PreparationofHydrophobicSiO2Aerogelsat-国防科技大学学报
三甲基硅烷化改性二氧化硅气凝胶

第21卷第1期强激光与粒子束Vol.21,N o.1 2009年1月H IGH POW ER LASER AND PA RTICLE BEAM S Jan.,2009 文章编号: 1001-4322(2009)01-0076-03三甲基硅烷化改性二氧化硅气凝胶*陈素芬, 李 波, 刘一杨, 张占文(中国工程物理研究院激光聚变研究中心,四川绵阳621900) 摘 要: 采用六甲基二硅氮烷对二氧化硅凝胶进行疏水处理,得到了疏水性的二氧化硅气凝胶。
用红外光谱和热分析表征处理前后二氧化硅气凝胶的性质,用测量显微镜跟踪处理前后气凝胶柱在空气中直径变化。
结果表明,处理后气凝胶的表面羟基明显减少,在空气中的吸潮性大大降低,圆柱体在空气中的径向收缩率从30%降至3%。
关键词: 二氧化硅; 气凝胶; 疏水处理; 三甲基硅烷化 中图分类号: T Q127.2; T L639.11 文献标志码: A 二氧化硅由于硅原子序数适中,并且易于制成低密度气凝胶,因此在激光惯性约束聚变(inertial confine-ment fusio n,ICF)研究中获得了广泛的应用,如辐射输运研究中的输运管填充材料[1-2]。
二氧化硅气凝胶网络表面存在羟基(-OH)和未水解的乙氧基(-OC2H5)官能团。
由于-OH的存在,气凝胶易吸收空气中的水分,导致网络结构塌陷,使得气凝胶收缩,其线性收缩率可达30%[3-5]。
而ICF辐射输运研究中的输运管长度只有几百μm,且要求填充材料均匀,输运管内部填充材料微小的收缩会使材料与管壁之间产生空隙,对最终的实验结果有很大的影响。
因此需要对二氧化硅气凝胶进行疏水改性,提高其对空气中水分的稳定性,降低其收缩率[6-7]。
文献报道,利用气态介质对气凝胶进行表面疏水处理,如气相甲氧基化,得到的气凝胶疏水性能很好,但是此方法成本高,工艺复杂,因此适用范围不广泛[8]。
此外,还可在合成前体时,在正硅酸甲酯(tetrame-tho xy silane,TM OS)中混入甲基三甲氧基硅烷(M eSi(OM e)3)[9],但是该凝胶在超临界干燥时易收缩,且随着M eSi(OMe)3用量增加,气凝胶的透明度降低。
Preparation-of-silica-aerogel-from-rice-hull-ash-by-supercritical-carbon-dioxide-drying

J.of Supercritical Fluids35(2005)91–94Preparation of silica aerogel from rice hull ash bysupercritical carbon dioxide dryingQi Tang,Tao Wang∗State Key Lab of Chemical Engineering,Department of Chemical Engineering,Tsinghua University,Beijing100084,ChinaReceived29September2004;accepted9December2004AbstractSilica aerogel,which is a mesoporous light solid material,was prepared from the rice hull ash by sol–gel followed supercritical carbon dioxide drying.The rice hull ash,which is rich in silica,was extracted using sodium hydroxide solution to produce a sodium silicate solution. The solution was neutralized with sulfuric acid solution to form a silica gel.After washing with water and the solvent exchange with ethanol, the aged gel was dried to produce aerogel using supercritical carbon dioxide drying.The prepared silica aerogel was characterized using SEM,TEM and BET measurements.The specific surface area of the rice hull ash aerogel was as high as597.7m2/g with a bulk density of 38.0kg/m3.The diameters of the pores inside the aerogel are between10and60nm.©2004Elsevier B.V.All rights reserved.Keywords:Supercritical drying;Rice hull ash;Silica aerogel1.IntroductionSilica aerogel is a highly porous,open cell,low-density foam.Since its microstructure consists of nano-sized pores and linked primary particles,it exhibits many desirable prop-erties,such as low thermal conductivity,good sound ab-sorbency and others associated with the refractive index, sound velocity and dielectric constant[1].The applications of the silica aerogel have expanded into manyfields[2]:(1)fillers for paints,varnishes,etc.;(2)thermal and acoustic in-sulation materials;(3)adsorbents and catalyst supports;(4) electronic materials such as Cerenkov detectors and sensor materials.The conventional method to prepare silica aerogel is sol–gel combined with supercritical drying.So far,much ef-fort has been devoted towards methods for the preparation of silica anic silicon monomers,such as tetram-ethylorthosilicate(TMOS),tetraethylorthosilicate(TEOS), and polyethoxydisiloxane(PEDS)are generally selected as precursors[3–5].However,such organic silicon precursors ∗Corresponding author.Tel.:+861062773017;fax:+861062770304.E-mail address:taowang@(T.Wang).are usually so expensive that silica aerogel production in an industrial scale is not economically practical.An important topic in aerogel research area is to explore cheap raw material for silica aerogel production.Rice hull ash,a waste of burning rice hull from the rice industry,is rich in silica.It could be used as a cheap silicon source.Kalapathy et al.[6]used rice hull ash as the raw material for production of pure silica.Yalcin and Sevinc[7] investigated to obtain relatively pure activated silica.In this work,rice hull ash was used as the silicon source for the preparation silica aerogel by a sol–gel method and followed supercritical CO2drying.2.Experimental2.1.MaterialsCO2(99.9%)was provided by Beijing Analytical Appa-ratus Co.(Beijing,China).Sulfuric acid(98%)and NaOH (95%)were provided by Beijing Chemical Reagent Research Institute.Ethanol(99%)was from Beijing Modern Oriental Fine Chemicals.De-ionized water was provided by Beijing0896-8446/$–see front matter©2004Elsevier B.V.All rights reserved. doi:10.1016/j.supflu.2004.12.00392Q.Tang,T.Wang /J.of Supercritical Fluids 35(2005)91–94Fig.1.Schematic procedure of preparing aerogel from rice hull ash.Agriculture University.All chemicals were used without any further purification.2.2.Preparation of silica aerogel from rice hull ash The procedure of preparing silica aerogel from rice hull ash was illustrated schematically in Fig.1.The rice hull was burned at 600◦C for 4h in an electric furnace to get rice hull ash.A 1.5g of the ash were mixed with 50mL 1mol/L NaOH aqueous solution.The mixture was heated up to its boiling point for 1.5h with the reflux.Then,the mixture was filtered to remove the undissolved residues.The filtrate was neutralized using 1mol/L sulfuric acid to pH =7to form silica hydrogel.The prepared gel was aged at room temperature for more than 24h.To remove the sodium sulfate resulted from the neutral-ization,the aged gel was washed using de-ionized water.Subsequently,the water in the silica gel was replaced by nonhydrous ethanol.After that,the pretreated silica gel was ready for the supercritical drying.Supercritical CO 2drying of the alcogel was conducted in a supercritical CO 2extraction system shown schematically in Fig.2.To be dried supercritically,the prepared silica gel sample of about 5cm 3volume was carefully put into the extraction autoclave,which had a volume of 50mL.The autoclave was pressurized with CO 2up to 16MPa at 25◦C for 24h.During this period,the ethanol in the silica gel was replaced by liquid CO 2.Then the autoclave was heated to 40◦C with constant pressure at 16MPa.Dynamic drying was performed with a CO 2flow rate of 1.5mol/h for 4h at 40◦C and 16MPa.After-wards,the autoclave was slowly depressurized to atmosphere at 40◦C.Finally,dried silica aerogel was obtained.2.3.Characterization of the aerogelDensity was measured by a mercury porosimeter (Micromertitics,Autopore IV 9500).BET surface areamea-Fig.2.Scheme of supercritical drying set-up.surements and pore distribution analysis were carried out with an Automatic Physorption and Chemsorption Analyzer (Micormeritics,Autosorb-1).Element analysis was mea-sured by ICP-OES (Leeman,Prodigy).Scanning electron microscopy (SEM)photography was performed on a SEM (Hitachi,S-450).Transmission electron microscopy (TEM)photography was performed on a TEM (Philips CM120).3.Results and discussionsThe prepared silica aerogel from rice hull ash was a very light,white porous bulk solid with an appearance as shown in Fig.3.The bulk density of this silica aerogel was as low as 38.0kg/m 3.From the bulk density of the aerogel and the density of pure amorphous silica [8],the porosity of this silica aerogel was estimated to be as 98.3%.The BET analysis results showed that the specific surface area was 597.7m 2/g,and total pore volume of pores was 8.65cm 3/g.The diameters of the pores in the aerogel are mainly distributed from 10to 60nm as shown in Fig.4.Unlike conventional organic silicon compounds,rice hull ash is a natural material,which contains many extraneous components besides silica.Elemental analysis of the rice hull ash and the aerogel obtained from it is given in Table 1.Table 1shows that the contents of the extraneous elements,except Na and S,were reduced to a low level during the aerogel preparation process.The increments of the contents ofNaFig.3.Silica aerogel with the preparation condition:1.5g rice hull ash and 50mL 1mol/L NaOH,boiling time 1.5h,neutralized with 1mol/L sulfuric acid to pH =7,aging time 24h,supercritical drying at 40◦C and 16MPa with CO 2flow rate of 1.5mol/h for 4h.Q.Tang,T.Wang/J.of Supercritical Fluids35(2005)91–9493Fig.4.Pore diameter distribution of the aerogel from rice hull ash.Prepa-ration conditions:same as ones in Fig.3.Table1Element comparison between rice hull ash and silica aerogelElement Rice hull ash(wt.%)Silica aerogel(wt.%) Al0.660.17Ca 1.290.015Fe0.440.063K 1.420.15Mg0.330.0076Mn0.0710.0011Na0.250.596P0.270.0056S0.230.376Zn0.0620.0085 Preparation conditions:same as ones in Fig.3.and S are due to the residue of sodium sulfate,which results from the neutralization in sol–gel steps and is difficult to be removed from the silica hydrogel.Scanning electron microscopy(SEM)photo(Fig.5)indi-cates that the prepared aerogel was a porous material with a continuous meshwork structure.In the SEM photo,the white parts are the bulges in the surface of the aerogel,while the black parts are pores in the aerogel.The transmission electron microscopy(TEM)photo(Fig.6)also confirmed that the pre-pared aerogel was a nanoporous material with the meshwork structure.The light parts in the TEM photo are the nanopores (1–50nm)inside the prepared aerogel.The characteristics of silica aerogels prepared from tetraethoxysilane(TEOS)and rice hull ash are comparedin Fig.5.The SEM photo of the silica aerogel from rice hull ash.Preparation conditions:same as ones in Fig.3.Fig.6.The TEM photo of the silica aerogel from rice hull ash.Preparation conditions:same as ones in Fig.3.Table2.The silica aerogel from rice hull ash and that from TEOS have comparable densities and pore volumes.How-ever,the specific area of the aerogel from rice hull ash was lower than that of the aerogel from TEOS.The white color of the aerogel from rice hull ash was different from the trans-parent color of the aerogel from TEOS.The result obtained by ambient pressure drying,is also listed in Table2.Although drying could be performed at ambient pressure,the procedure gives a xerogel instead ofTable2Comparison between silica aerogels prepared with different precursors and drying methodsPrecursor/drying method Color Density(kg/m3)BET surface area(m2/g)Pore volume(cm3/g) TEOS/sc-ethanol drying a[9]Transparent43.0–89.0935–11508.6–10.9Rice hull ash/scCO2drying b White38.0597.78.7Rice hull ash/ambient pressure drying c Semitransparent1716.7 4.40.1a Result from Ref.[9].b Preparation conditions:same as ones in Fig.3.c Preparation conditions:drying at ambient pressure and100◦C for10h,others are same as ones in Fig.3.94Q.Tang,T.Wang/J.of Supercritical Fluids35(2005)91–94an aerogel.During drying of the gel under ambient pres-sure,capillary pressure causes shrinkage and cracking of the gel network,so that the produced xerogel has high den-sity,very low specific area and pore volume.Shrinkage of a gel during drying is driven by the capillary pressure,which can be reduced by reducing surface tension of the pore liq-uid[10].Supercritical drying eliminates surface tension,and keeps the structure of the gel from destroying to obtain the aerogel.4.ConclusionRice hull ash could be used as the precursor of silica aero-gel.The silica aerogel obtained by supercritical drying of a gel from rice hull ash using sol–gel is a lightweight meso-porous solid material with a bulk density as low as38.0kg/m3 and porosity as high as98.3%.The silica aerogel from the rice hull ash obtained by supercritical drying had narrowly distributed pores from10to60nm,and a BET surface area of597.7m2/g and a pore volume of8.65cm3/g.References[1]J.Fricke,T.Tillotson,Aerogels:production,characterization,andapplications,Thin Solid Films297(1997)2123.[2]M.Schmidt,F.Schwertfeger,Applications for silica aerogel prod-ucts,J.Non-Crystalline Solids225(1998)364.[3]S.Yoda,S.Ohshima,F.Ikazaki,Supercritical drying with zeolitefor the preparation of silica aerogels,J.Non-Crystalline Solids231 (1998)41.[4]H.Tamon,T.Kitamura,M.Okazaki,Preparation of silica aerogelfrom TEOS,J.Colloid Interface Sci.197(1998)353.[5]A.V.Rao,M.M.Kulkarni,D.P.Amalnerkar,T.Seth,Surface chemi-cal modification of silica aerogels using various alkyl-alkoxy/chloro silanes,Appl.Surf.Sci.206(2003)262.[6]U.Kalapathy,A.Proctor,J.Shultz,A simple method for productionof pure silica from rice hull ash,Bioresour.Technol.73(2000)257.[7]N.Yalcin,V.Sevinc,Studies on silica obtained from rice husk,Ceramics Int.27(2001)219.[8]R.C.Weast,CRC Handbook of Chemistry and Physics,63rd ed.,CRC Press,1983,p.B143.[9]M.Stolarski,J.Walendziewski,M.Steininger,B.Pniak,Synthesisand characteristic of silica aerogels,Appl.Catal.A:Gen.177(1999) 139.[10]C.J.Brinker,G.W.Scherer,Sol–gel Science:The Physics and Chem-istry of Sol–gel Processing,Academic Press,New York,1990.。
原位法常压干燥制备疏水SiO_2气凝胶及其热稳定性
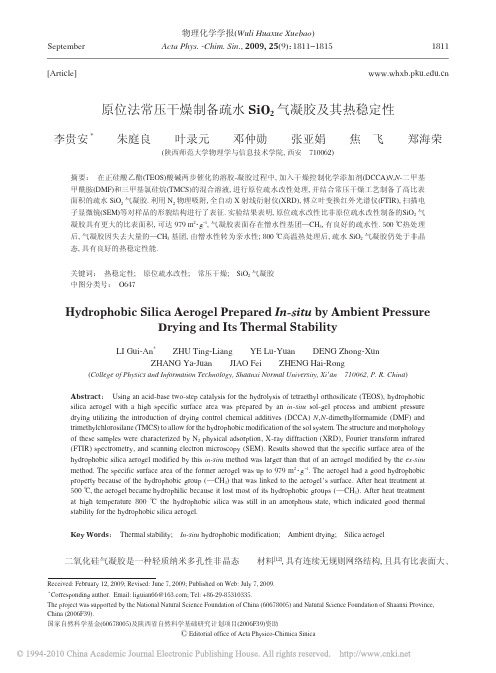
2.1 气凝胶的物相及形貌分析 图 1 为原位法制备的疏水 SiO2 气凝胶的 XRD
图谱. 由图 1 可知, 所得疏水 SiO2 气凝胶样品仅在 2θ 为 20°-25°范围内有一个馒头状弥散宽峰, 在其 它位置没有明显的特征衍射峰, 说明该样品是典型 的无定型非晶态结构.
图 2 为疏水 SiO2 气凝胶的扫描电镜照片. 由图 2 可知, 气凝胶表面呈不规则颗粒紧簇分布状, 有少 量大颗粒. 2.2 气凝胶的 FTIR 分析
(College of Physics and Information Technology, Shaanxi Normal University, Xi′an 710062, P. R. China)
Abstract: Using an acid-base two-step catalysis for the hydrolysis of tetraethyl orthosilicate (TEOS), hydrophobic silica aerogel with a high specific surface area was prepared by an in-situ sol-gel process and ambient pressure drying utilizing the introduction of drying control chemical additives (DCCA) N,N-dimethylformamide (DMF) and trimethylchlorosilane (TMCS) to allow for the hydrophobic modification of the sol system. The structure and morphology of these samples were characterized by N2 physical adsorption, X-ray diffraction (XRD), Fourier transform infrared (FTIR) spectrometry, and scanning electron microscopy (SEM). Results showed that the specific surface area of the hydrophobic silica aerogel modified by this in-situ method was larger than that of an aerogel modified by the ex-situ method. The specific surface area of the former aerogel was up to 979 m2·g-1. The aerogel had a good hydrophobic property because of the hydrophobic group (—CH3) that was linked to the aerogel′s surface. After heat treatment at 500 ℃, the aerogel became hydrophilic because it lost most of its hydrophobic groups (—CH3). After heat treatment at high temperature 800 ℃ the hydrophobic silica was still in an amorphous state, which indicated good thermal stability for the hydrophobic silica aerogel.
十七氟癸基修饰的有机-无机杂化二氧化硅膜材料的制备及气体分离性能

十七氟癸基修饰的有机-无机杂化二氧化硅膜材料的制备及气体分离性能宋霖;韦奇;郝润秋;聂祚仁;李群艳【摘要】以十七氟癸基三乙氧基硅烷( PFDTES)和1,2-双(三乙氧基硅基)乙烷(BTESE)为前驱体,通过溶胶-凝胶法制备了十七氟癸基修饰的SiO2溶胶,采用浸渍提拉法在γ-Al2 O3/α-Al2O3多孔陶瓷支撑体上涂膜,然后在N2气氛保护下烧结成完整无缺陷的有机-无机杂化SiO2膜.利用扫描电子显微镜对膜材料的形貌进行观察,通过动态光散射技术对溶胶粒径及分布进行测试,利用视频光学接触角测量仪、红外光谱仪和热分析仪表征了十七氟癸基修饰对有机-无机杂化SiO2膜疏水性的影响.结果表明,十七氟癸基已经成功修饰到SiO2膜材料中,且随着PFDTES加入量的增大,溶胶粒径和膜材料对水的接触角不断增大.当n(PFDTES)∶n(BTESE)=0.25∶1时,溶胶粒径分布较窄,平均粒径为3.69 nm,膜材料对水的接触角为(112.0±0.4)°.在修饰后的有机-无机杂化SiO2膜中H2的输运遵循微孔扩散机理,在300℃时,H2的渗透率达到5.99×10-7mol·m-2·Pa-1·s-1,H2/CO和H2/CO2的理想分离系数分别达到9.54和5.20,均高于Knudsen扩散的理想分离因子,表明膜材料具有良好的分子筛分效应.%Hydrophobic organic-inorganic hybrid silica sols were prepared using 1,2-bis(triethoxysilyl) ethane (BTESE) and 1H,1H,2H,2H-perfluorodecyltriethoxysilane(PFDTES) as precursors under acidic condition, then the sols were deposited on γ-Al2O3/α-Al2O3 substrates by dip-coating under clean room condition. The cross-sectional morphology, particle size of the sols and hydrophobic property of the membranes were characterized by means of SEM, DLS, FTIR, water contact angle measurement and thermogravimetric analysis.The results show that the particle size of sols and the water contact angle of supported membranes increase with increasing the amount of PFDTES in the mixture. A narrow sol particle size distribution centered at 3. 69 nm and a water contact angle of (112.0±0.4) ° were obtained at aBTESE/PFDTES molar ratio of 0.25: 1. At 300 ℃, the membr ane shows a high H2 permeance of 5. 99×10-7mol·m-2·Pa-1·s-1 and a H2/CO andH2/CO2 permselectivity of 9. 54 and 5. 20, respectively, which were higher than the corresponding Knudsen value(H2/ CO=3.74, H2/CO2=4. 69). The H2 transport in the modified silica membranes is subjected to a micropore diffusion mechanism.【期刊名称】《高等学校化学学报》【年(卷),期】2012(033)008【总页数】6页(P1670-1675)【关键词】有机-无机杂化SiO2膜;溶胶-凝胶法;疏水性;气体分离【作者】宋霖;韦奇;郝润秋;聂祚仁;李群艳【作者单位】北京工业大学材料科学与工程学院,北京100124;北京工业大学材料科学与工程学院,北京100124;北京工业大学材料科学与工程学院,北京100124;北京工业大学材料科学与工程学院,北京100124;北京工业大学材料科学与工程学院,北京100124【正文语种】中文【中图分类】O613.7氢气分离技术是化石燃料制氢工艺中最重要的步骤之一.膜分离法具有投资少、能耗低、装置及操作简单、可在温和条件下实现连续分离及对环境友好等优点[1~3],极具发展前景.用于氢气分离的膜材料主要有分子筛膜、金属Pd膜和微孔陶瓷膜等,其中无定形的微孔SiO2膜被认为是最能接近工业应用的氢气分离材料之一[4~7].但SiO2膜的水热稳定性差,长期暴露于水热环境下会发生分解反应,从而导致膜破裂形成缺陷,影响气体分离效果[8].为了提高SiO2膜的水热稳定性,众多研究者开展了大量工作:(1)将过渡金属离子掺杂到SiO2骨架中以提高膜材料的稳定性,因为氧原子与过渡金属形成的共价键比硅氧键更稳定[9,10];(2)通过化学修饰在SiO2膜表面引入疏水基团,提高膜材料的疏水性,减少水分子在膜材料表面的吸附,降低水分子对Si—O—Si结构的破坏.1999年,de Vos等[11]首次将甲基修饰到SiO2膜表面,使其疏水性能得到显著提高.而后Wei等[12]制备了三氟丙基修饰的SiO2膜,在努力提高SiO2膜渗透率和分离系数的同时也提高了其抵抗水汽的性能.上述SiO2膜材料都是以正硅酸乙酯(TEOS)为前驱体制备的,膜材料的骨架依然是—Si—O—Si—网络结构,由于这种结构在水热环境下易水解,导致所制备的SiO2膜的长期水热稳定性不够理想.用1,2-双(三乙氧基硅基)乙烷(BTESE)作为前驱体制备有机-无机杂化SiO2膜,基于BTESE的膜材料骨架主要由—Si—C—C—Si—结构组成,与—Si—O—Si—结构相比,在水热环境下不会发生水解反应而导致膜材料产生缺陷.Kanezashi等[13]以BTESE为前驱体,在以α-Al2O3为基体、SiO2-ZrO2为过渡层的管式载体表面涂膜,于300℃焙烧30 min后得到具有较高He和H2渗透通量的有机-无机杂化SiO2膜.Castricum等[14,15]分别以甲基三乙氧基硅烷(MTES)和TEOS以及MTES和BTESE为前驱体制备了SiO2膜,结果显示,基于BTESE的有机-无机杂化SiO2膜与传统无机SiO2膜及甲基化SiO2膜相比,在正丁醇脱水的渗透汽化环境下,水热稳定性得到显著提高.尽管基于BTESE的有机-无机杂化SiO2膜比基于TEOS的无机SiO2膜具有更高的水热稳定性,但BTESE的水解缩聚反应过程也会在膜材料骨架中引入少量的—Si—O—Si—结构,加上有机-无机杂化SiO2膜还具有一定的亲水性[16],因而在水热环境下膜中的—Si—O—Si—结构还有可能受到水分子的攻击.为了减小水分子对有机-无机杂化SiO2膜的破坏,本文通过溶胶修饰的方法,用十七氟癸基三乙氧基硅烷(PFDTES)代替部分BTESE作为共前驱体,经共水解缩合反应制备有机-无机杂化SiO2膜,以提高膜的疏水性能,并研究了十七氟癸基修饰对溶胶结构的影响以及修饰后有机-无机杂化SiO2膜的氢气渗透和分离性能.1.1 实验原料1,2-双(三乙氧基硅基)乙烷(BTESE),分析纯,Sigma-Aldrich化学有限公司;十七氟癸基三乙氧基硅烷(PFDTES),分析纯,Alfa Aesar化学有限公司;硝酸,优级纯,广东汕头市西陇化工厂;无水乙醇(EtOH),分析纯,北京化工厂;去离子水,由优普ULUP-Ⅰ-10T型超纯水机制备.1.2 有机-无机杂化SiO2溶胶及膜材料的制备首先将BTESE和PFDTES在冰水浴中搅拌,使其混合均匀,然后加入无水乙醇继续搅拌,最后逐滴加入事先按比例混合好的浓硝酸和蒸馏水,再继续搅拌一段时间后,将此混合物于60℃水浴中剧烈搅拌3 h,最终混合物的摩尔比为n(BTESE)∶n(PFDTES)∶n(EtOH)∶n(H2O)∶n(HNO3)=1∶x∶10.5∶5.25∶0.1(x =0,0.11,0.25和0.43),反应结束后得到无色透明的十七氟癸基修饰的有机-无机杂化SiO2溶胶.将新制备的溶胶用无水乙醇以1∶19(体积比)稀释,然后通过浸渍提拉法(Dip-coating)将其涂覆在γ-Al2O3/α-Al2O3多孔陶瓷支撑体上.为了避免空气微颗粒在膜材料表面形成缺陷,整个涂膜过程在100级洁净室中进行.涂膜后于380℃煅烧4 h,为了防止氧化,在煅烧过程中以N2作为保护气.为了得到无缺陷的SiO2膜,上述涂膜和煅烧过程需重复4次.将剩余的SiO2溶胶干燥成凝胶,然后在380℃及N2气氛下煅烧4 h,得到无支撑的十七氟癸基修饰的有机-无机杂化SiO2膜.修饰的SiO2膜样品根据PFDTES的化学计量标记为(xPFDTES)BTESE(x=0,0.11,0.25和0.43).1.3 有机-无机杂化SiO2膜的表征有支撑有机-无机杂化SiO2膜的断面和表面形貌用JEOL JSM-7001F型扫描电镜观察.采用OCA20视频光学接触角测量仪(德国Dataphysics公司)测量膜材料的接触角(CA),室温下,将体积为2 μL的水滴以1 μL/s的速度滴在有支撑SiO2膜的表面,然后测量水滴在膜材料表面的接触角.热重分析在STA449C/1/G型热分析仪(德国耐驰公司)上进行,将(0PFDTES)BTESE样品和(0.25PFDTES)BTESE样品放入相对湿度为70%~80%、温度为40℃的水热环境中分别陈化20和40 d后进行热重分析,在30 mL/min的N2气吹扫下,以10℃/min的升温速度从室温加热到650℃.利用Nicolet 5700型傅里叶变换红外光谱仪(美国热电公司)测定(0PFDTES)BTESE和(0.25PFDTES)BTESE样品的红外光谱.通过动态光散射技术(DLS),在Zetasizer Nano ZS 90型激光粒度仪(英国马尔文公司)上进行溶胶粒径及分布测试.H2的渗透和分离性能测试在自制的气体渗透和分离装置上完成,有支撑有机-无机杂化SiO2膜采用柔性石墨垫片密封,膜材料两端的压力差用CWY100精密数字压力表(西安创威科技公司)测量,调节稳压阀使得膜材料两侧压差为0.1 MPa,随后加热渗透装置,待温度恒定后用电子气体流量计(Alicat Scientific Inc.公司)测量气体的质量流量.2.1 有支撑有机-无机杂化SiO2膜的形貌图1为有支撑有机-无机杂化SiO2膜的断面和表面的SEM照片.从图1(A)可见,膜体系主要由三层组成:顶层为有机-无机杂化SiO2膜,厚度约170 nm;中间为γ-Al2O3过渡层;底层为α-Al2O3多孔陶瓷.这说明有机-无机杂化SiO2膜已浸涂到γ-Al2O3/α-Al2O3衬体上.由图1(B)可见,该膜材料的表面平整光洁,无明显的裂纹和大孔等严重缺陷.2.2 有支撑有机-无机杂化SiO2膜的疏水性能图2是水滴在有支撑有机-无机杂化SiO2膜表面的照片.由图2可见,水滴在未修饰膜表面的接触角只有(49.0±0.5)°[图2(A)],随着PFDTES加入量的增大,接触角不断增大[图2(B)~(D)],且膜材料由亲水变成疏水,这是由于膜表面的部分羟基被疏水的十七氟癸基取代所致.从图2(C)可见,当n(PFDTES)∶n(BTESE)=0.25∶1时,接触角达到(112.0±0.4)°,与文献[17]中制备的三氟丙基修饰SiO2膜、文献[18]中制备的苯基修饰SiO2膜和文献[19]中制备的乙烯基修饰SiO2膜进行对比发现,当达到相同的接触角时,本文所需要修饰的十七氟癸基的量比三氟丙基、苯基和乙烯基少很多,说明修饰十七氟癸基比修饰三氟丙基、苯基和乙烯基具有更强的疏水效果.从图2(C)和(D)中还可以看出,水滴在(0.25PFDTES)BTESE和(0.43PFDTES)BTESE表面上的接触角分别为(112.0±0.4)°和(112.7±0.9)°,两者相差无几.这是因为修饰量达到一定程度后,修饰到膜材料表面的十七氟癸基密度增大,由于十七氟癸基是长链基团,占据较大的空间位阻,所以即使修饰量增大,十七氟癸基也较难取代更多的表面羟基,因而膜材料的疏水性能未能进一步提高.图3是无支撑有机-无机杂化SiO2样品在水热环境(温度40℃、相对湿度70% ~80%)处理前后的热重分析结果.可见,(0PFDTES)BTESE膜材料陈化20和40 d后有明显的失重,失重量分别为8%和10%,且随着陈化时间的延长,失重量逐渐增加.失重开始温度为50℃左右,这是物理吸附水的脱附引起的,到150℃左右这一阶段失重基本完成.而未陈化的(0PFDTES)BTESE膜材料在50~150℃基本未失重,说明在湿热环境的陈化过程中,膜材料表面未反应的硅醇悬键将水蒸气吸附在膜的表面,这会严重影响膜的结构和性能,导致孔径发生变化,从而影响气体分离效果[20,21]. 而(0.25PFDTES)BTESE膜材料无论是未陈化还是陈化20 d或40 d,在50~150℃基本没有失重,表明用疏水基团(十七氟癸基)修饰的膜材料在湿热环境下基本没有吸附水蒸气.与(0PFDTES)BTESE膜材料的失重行为相比,(0.25PFDTES)BTESE膜材料展现了优异的疏水性能.图4为修饰前后的有机-无机杂化SiO2膜的FTIR谱图.其中1048 cm-1处的吸收峰为Si—O—Si伸缩振动的特征峰,3455 cm-1处为物理吸附水的吸收峰,1628 cm-1处的吸收峰对应表面羟基Si—OH.对于十七氟癸基修饰的样品,1248和1150 cm-1处的吸收峰对应于C—F的振动峰,而未修饰的SiO2样品在上述波数处均未出现吸收峰,说明十七氟癸基已经成功修饰到SiO2膜材料中,且经过380℃的煅烧,C—F键并没有被破坏.从图4还可看出,未修饰的SiO2膜在3455 cm-1处归属于物理吸附水的吸收峰非常强烈,说明未修饰的膜材料疏水性较差;而十七氟癸基修饰后的膜材料吸附水的吸收峰则较弱,说明经修饰后膜材料的疏水性得到增强.同时还可以看出,随着PFDTES的加入,膜材料中Si—OH在1628 cm-1处的吸收峰明显减弱,表明Si—OH浓度逐渐降低,这是由于十七氟癸基取代膜材料中的部分羟基,增强了膜材料的疏水性能,这与图2接触角测量结果一致.2.3 溶胶粒径及分布基于BTESE的有机-无机杂化SiO2膜材料的结构主要由溶胶-凝胶反应所得的低聚物经过进一步组合而成,所谓的溶胶胶粒指的即是这些由Si—C—C—Si结构单元组成的低聚物.PFDTES的分子结构上存在一个没有反应活性的基团,即十七氟癸基,当BTESE和PFDTES进行共水解缩聚反应形成溶胶时,它并不参与反应,其结果是这些基团与硅原子形成悬键伸出低聚物的外表面,形成所谓的“分支”,低聚物通过这些分支的相互穿插,即可得到膜材料的微孔结构,因而这些低聚物的尺寸即溶胶胶粒的粒径对BTESE膜材料的孔结构起到重要的调控作用.本文利用十七氟癸基的修饰来提高膜材料的疏水性,必然对溶胶胶粒产生影响,因此必须综合考虑十七氟癸基对膜材料的疏水性及溶胶胶粒的影响.图5是不同修饰量的有机-无机杂化SiO2溶胶的粒径分布图.由图5可见,(0.11PFDTES)BTESE的粒径较小(平均1.74 nm),且粒径分布较窄(1.50~2.01 nm);随着PFDTES加入量的增大,SiO2溶胶的平均粒径逐渐增大,粒径分布也逐渐变宽;(0.43PFDTES)BTESE的平均粒径达到了12.73 nm,粒径分布也较宽(8.72~18.17 nm).这是因为十七氟癸基的分子链较长,当修饰量增大时,修饰到—Si—C—C—Si—网络结构上致使整个溶胶粒径变大.选择溶胶进行涂膜时,溶胶粒径大小最好与γ-Al2O3/α-Al2O3多孔陶瓷支撑体的孔径(约4 nm)相近,因为选择粒径大的溶胶(>10 nm)进行涂膜会得到较厚的SiO2膜层,导致膜层易形成裂纹或其它严重缺陷,从而影响膜层的分离效果[22].另外,过大的溶胶胶粒必然使得膜材料的孔径过大,不利于气体分子的分离;而粒径过小时,溶胶颗粒可能直接进入γ-Al2O3/α-Al2O3支撑体的孔隙中,也会使膜层形成缺陷.由图5可见,(0.25PFDTES)BTESE溶胶的平均粒径为3.69 nm,且粒径分布较窄,疏水性能也较好[对水的接触角为(112.0±0.4)°],因此本文选择(0.25PFDTES)BTESE溶胶涂膜并进行气体分离实验.2.4 单组分气体渗透和分离性能图6为(0.25PFDTES)BTESE膜体系在不同温度下H2,CO2,CO和SF6的渗透率,表1为不同温度下H2/CO,H2/CO2,H2/SF6,CO/SF6和CO2/SF6的理想分离系数及其 Knudsen扩散分离因子.从图6和表1可见,H2的渗透率随着温度的升高逐渐增大,300℃时达到5.99×10-7mol·m-2·Pa-1·s-1;H2/CO2,H2/CO和H2/SF6的理想分离系数分别达到了5.20,9.54和39.08,均高于各自的Knudsen扩散分离因子(H2/CO2=4.69,H2/CO=3.74,H2/SF6=8.54),说明H2在膜材料中的输运行为主要遵循微孔扩散机理,且膜材料在此温度下对H2/CO2,H2/CO和H2/SF6的分离起到了分子筛分的作用.H2,CO2,CO和SF6的分子动力学直径分别为0.289,0.33,0.376和0.55 nm,SF6只能在尺寸大于0.55 nm的微孔或介孔甚至微缺陷中输运.从图6可以看出,SF6的渗透率随着温度的升高而降低,说明SF6的输运受到Knudsen扩散机制的控制.而H2,CO2和CO气体分子既能进入到小于0.55 nm的微孔中输运,也能在大于0.55 nm的微孔或介孔甚至微缺陷中输运,因而理论上CO和CO2气体的输运既可能遵循微孔扩散机理,也可能受Knudsen扩散机制的控制.从图6可见,CO2和CO的渗透率随着温度的升高而降低,其气体分子的输运呈现出Knudsen扩散现象,然而从表1可见,在不同温度时CO/SF6和CO2/SF6的分离系数均大于各自的Knudsen扩散分离因子,这说明CO和CO2气体的输运确实也受到微孔扩散机理的控制.在温度为50℃时,膜材料对于H2/CO的理想分离系数低于其Knudsen扩散分离因子;在温度为50~250℃时,膜材料对于H2/CO2的理想分离系数低于其Knudsen扩散分离因子.这可能是由于在此温度下CO和CO2的输运主要受到Knudsen扩散机制的控制,导致其在温度降低时渗透率增加,从而使得H2/CO和H2/CO2的理想分离系数降低.综上所述,通过十七氟癸基三乙氧基硅烷(PFDTES)和1,2-双(三乙氧基硅基)乙烷(BTESE)的共水解缩聚反应可得到稳定的有机-无机杂化SiO2溶胶,溶胶的粒径随着PFDTES修饰量的增加而增大.当n(PFDTES)∶n(BTESE)=0.25∶1时,溶胶的平均粒径为3.69 nm,分布范围较窄,用此溶胶在γ-Al2O3/α-Al2O3多孔陶瓷支撑体上涂覆的有机-无机杂化SiO2膜[(0.25PFDTES)BTESE]对水的接触角可达(112.0±0.4)°.300 ℃时该膜材料的 H2 渗透率达到5.99×10-7mol·m-2·Pa-1·s-1,H2/CO2,H2/CO和H2/SF6的分离系数分别为5.20,9.54和39.08,均高于Knudsen扩散的理想分离因子,表现出良好的分子筛分效应.[1] Kawi S.,Lai mun.[J],1998,13:1047—1053 [2] Anita R.,Sunil A.,Yang S.S..Renew.Energ.[J],2010,35:2649—2655[3] Zahmakiran M.,Ayvali T.,Akbayrak S.,Cali?kan S.,elik D.,Ozkar S..Catalysis Today[J],2011,170(1):76—84[4] Araki S.,Imasaka S.,Tanaka S.,Miyake Y..J.Membr.Sci.[J],2011,380:41—47[5] Catalano J.,Baschetti M.G.,Sarti G.C..Int.J.Hydrogen.Energ.[J],2011,36(14):8658—8673[6] Pomerantz N.,Ma Y.H..J.Membr.Sci.[J],2011,370:97—108 [7] Varela-Gandia F.J.,Berenguer-Murcia A.,Lozano-Castello D.,Cazorla-Amoros D..J.Membr.Sci.[J],2011,378:407—414[8] Imai H.,Morimoto H.,Tominaga A.,Hirashima H..J.Sol-GelSci.Technol.[J],1997,10:45—54[9] Uhlmann D.,Liu S.,Ladewing B.P.,Diniz da Costa J.C..J.Membr.Sci.[J],2009,326(2):316—321[10] WEI Na-Na(魏娜娜),WEI Qi(韦奇),LI Zhen-Jie(李振杰),LI Qun-Yan(李群艳),NIE Zuo-Ren(聂祚仁).J.Inorg.Mater.(无机材料学报)[J],2010,25(10),1047—1052[11] de Vos R.M,Wilhelm F.M.,Henk V..J.Membr.Sci.[J],1999,158:277—278[12] Wei Q.,Wang F.,Nie Z.R.,Song C.L.,Wang Y.L.,LiQ.Y..J.Phys.Chem.B[J],2008,112:9354—9359[13] Kanezashi M.,Yada K.,Yoshioka T.,Tsuru T..J.Membr.Sci.[J].2010,348:310—318[14] Castricum H.L.,Sah A.,Kreiter R.,Blank D.H.A.,Vente J.F.,ten Elshof J.E..J.Mater.Chem.[J],2008,18:2150—2158[15] Castricum H.L.,Sah A.,Geenevasen J.A.J.,Kreiter R.,BlankD.H.A.,Vente J.F.,ten Elshof J.E..J.Sol-Gel.Sci.Technol.[J],2008,48:11—17[16] DUAN Xiao-Yong(段小勇),WEI Qi(韦奇),HE Jun(何俊),LI Qun-Yan(李群艳),NIE Zuo-Ren(聂祚仁).Chem.J.Chinese Universities(高等学校化学学报)[J],2011,32(10):2256—2261[17] WANG Fei(王飞),WEI Qi(韦奇),WANG Yan-Li(王艳丽),YU Chun-Xiao(于春晓),ZHONG Zhen-Xing(钟振兴),LI Qun-Yan(李群艳),NIE Zuo-Ren(聂祚仁).Acta Chim.Sin.(化学学报)[J],2008,66(1):44—48[18] LI Zhen-Jie(李振杰),WEI Qi(韦奇),WEI Na-Na(魏娜娜),LI Qun-Yan(李群艳),NIE Zuo-Ren(聂祚仁).Chem.J.Chinese Universities(高等学校化学学报)[J],2010,31(12):2482—2487[19] Wei Q.,Wang Y.L.,Nie Z.R.,Yu C.X.,Li Q.Y.,Zou J.X.,LiC.J..Microp.Mesop.Mater.[J],2008,11:97—103[20] Turov V.V.,Mironyuk I.F..Colloid Surface A[J],1998,134:257—263[21] WEI Qi(韦奇),LI Jian-Lin(李建林),SONG Chun-Lin(宋春林),LIU Wei(刘卫),CHEN Chu-Sheng(陈初升).J.Inorg.Mater.(无机材料学报)[J],2004,19(2):417—423[22] Castricum H.L.,Sah A.,Kreiter R.,Blank D.H.A.,Vente J.F.,ten Elshof mun.[J],2008,9:1103—1105【相关文献】[1] Kawi S.,Lai mun.[J],1998,13:1047—1053[2] Anita R.,Sunil A.,Yang S.S..Renew.Energ.[J],2010,35:2649—2655[3] Zahmakiran M.,Ayvali T.,Akbayrak S.,Cali?kan S.,elik D.,Ozkar S..Catalysis Today[J],2011,170(1):76—84[4] Araki S.,Imasaka S.,Tanaka S.,Miyake Y..J.Membr.Sci.[J],2011,380:41—47 [5] Catalano J.,Baschetti M.G.,Sarti G.C..Int.J.Hydrogen.Energ.[J],2011,36(14):8658—8673[6] Pomerantz N.,Ma Y.H..J.Membr.Sci.[J],2011,370:97—108[7] Varela-Gandia F.J.,Berenguer-Murcia A.,Lozano-Castello D.,Cazorla-Amoros D..J.Membr.Sci.[J],2011,378:407—414[8] Imai H.,Morimoto H.,Tominaga A.,Hirashima H..J.Sol-Gel Sci.Technol.[J],1997,10:45—54[9] Uhlmann D.,Liu S.,Ladewing B.P.,Diniz da Costa J.C..J.Membr.Sci.[J],2009,326(2):316—321[10] WEI Na-Na(魏娜娜),WEI Qi(韦奇),LI Zhen-Jie(李振杰),LI Qun-Yan(李群艳),NIE Zuo-Ren(聂祚仁).J.Inorg.Mater.(无机材料学报)[J],2010,25(10),1047—1052[11] de Vos R.M,Wilhelm F.M.,Henk V..J.Membr.Sci.[J],1999,158:277—278 [12] Wei Q.,Wang F.,Nie Z.R.,Song C.L.,Wang Y.L.,Li Q.Y..J.Phys.Chem.B[J],2008,112:9354—9359[13] Kanezashi M.,Yada K.,Yoshioka T.,Tsuru T..J.Membr.Sci.[J].2010,348:310—318[14] Castricum H.L.,Sah A.,Kreiter R.,Blank D.H.A.,Vente J.F.,ten ElshofJ.E..J.Mater.Chem.[J],2008,18:2150—2158[15] Castricum H.L.,Sah A.,Geenevasen J.A.J.,Kreiter R.,Blank D.H.A.,Vente J.F.,ten Elshof J.E..J.Sol-Gel.Sci.Technol.[J],2008,48:11—17[16] DUAN Xiao-Yong(段小勇),WEI Qi(韦奇),HE Jun(何俊),LI Qun-Yan(李群艳),NIE Zuo-Ren(聂祚仁).Chem.J.Chinese Universities(高等学校化学学报)[J],2011,32(10):2256—2261[17] WANG Fei(王飞),WEI Qi(韦奇),WANG Yan-Li(王艳丽),YU Chun-Xiao(于春晓),ZHONG Zhen-Xing(钟振兴),LI Qun-Yan(李群艳),NIE Zuo-Ren(聂祚仁).Acta Chim.Sin.(化学学报)[J],2008,66(1):44—48[18] LI Zhen-Jie(李振杰),WEI Qi(韦奇),WEI Na-Na(魏娜娜),LI Qun-Yan(李群艳),NIE Zuo-Ren(聂祚仁).Chem.J.Chinese Universities(高等学校化学学报)[J],2010,31(12):2482—2487[19] Wei Q.,Wang Y.L.,Nie Z.R.,Yu C.X.,Li Q.Y.,Zou J.X.,LiC.J..Microp.Mesop.Mater.[J],2008,11:97—103[20] Turov V.V.,Mironyuk I.F..Colloid Surface A[J],1998,134:257—263[21] WEI Qi(韦奇),LI Jian-Lin(李建林),SONG Chun-Lin(宋春林),LIU Wei(刘卫),CHEN Chu-Sheng(陈初升).J.Inorg.Mater.(无机材料学报)[J],2004,19(2):417—423[22] Castricum H.L.,Sah A.,Kreiter R.,Blank D.H.A.,Vente J.F.,ten Elshof mun.[J],2008,9:1103—1105(Ed.:N,K,M)。
水解法制备纳米二氧化硅和表征_庞久寅
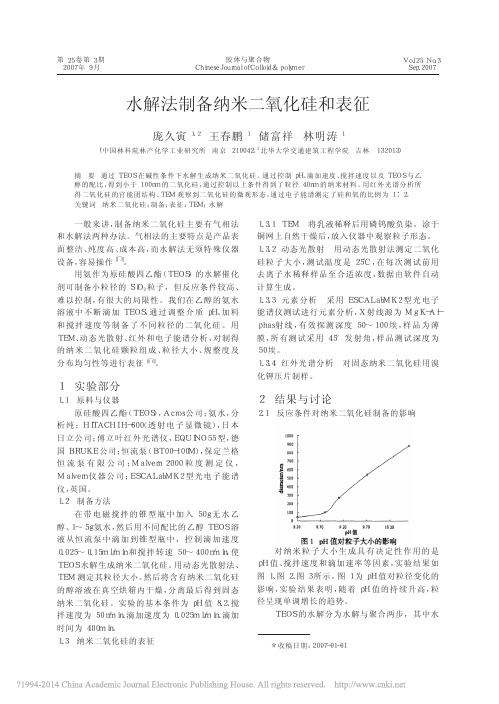
摘 要 通过 TEOS 在碱性条件下水解生成纳米二氧化硅。通过控制 pH、滴加速度、搅拌速度以及 TEOS 与乙 醇的配比, 得到小于 100nm 的二氧化硅, 通过控制以上条件得到了粒径 40nm 的纳米材料。用红外光谱分析所 得二氧化硅的官能团结构、TEM 观察到二氧化硅的微观形态, 通过电子能谱测定了硅和氧的比例为 1∶2。 关键词 纳米二氧化硅; 制备; 表征; TEM; 水解
在 带 电 磁 搅 拌 的 锥 型 瓶 中 加 入 50g 无 水 乙 醇、1~5g 氨水, 然后用不同配比的乙醇 TEOS 溶 液从恒流泵中滴加到锥型瓶中, 控制滴加速度 0.025~0.15ml/min 和搅拌转速 50~400r/min, 使 TEOS 水解生成纳米二氧化硅。用动态光散射法、 TEM 测定其粒径大小。然后将含有纳米二氧化硅 的醇溶液在真空烘箱内干燥, 分离最后得到固态 纳米二氧化硅。实验的基本条件为 pH 值 8.2、搅 拌速度为 50r/min、滴加速度为 0.025ml/min、滴加 时间为 400min。 1.3 纳米二氧化硅的表征
2 结果与讨论
2.1 反应条件对纳米二氧化硅制备的影响
pH 值
图 1 pH 值对粒子大小的影响 对纳米粒子大小生成具有决定性作用的是
pH 值、搅拌速度和滴加速率等因素, 实验结果如 图 1、图 2、图 3 所示。图 1 为 pH 值对粒径变化的 影响, 实验结果表明, 随着 pH 值的持续升高, 粒 径呈现单调增长的趋势。
图 6b。 2.4 元素分析
通过图 7 可以看出, 通过对二氧化硅粉末的 XPS 测定可以看出图中只有硅和氧的吸收峰, 且 两 者 的 面 积 比 为 1 ∶2, 因 而 断 定 其 分 子 式 为 SiO2。
SiO2气凝胶壳聚糖复合药物载体材料的制备和表征
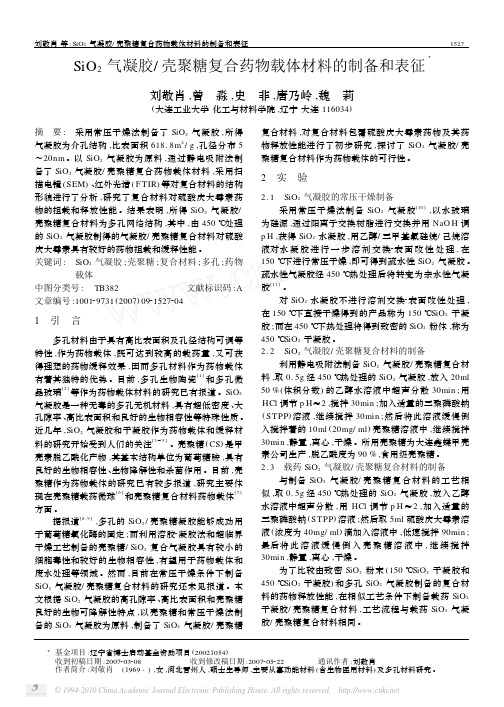
SiO2气凝胶/壳聚糖复合药物载体材料的制备和表征3刘敬肖,曾 淼,史 非,唐乃岭,魏 莉(大连工业大学化工与材料学院,辽宁大连116034)摘 要: 采用常压干燥法制备了SiO2气凝胶,所得气凝胶为介孔结构,比表面积618.8m2/g,孔径分布5~20nm。
以SiO2气凝胶为原料,通过静电吸附法制备了SiO2气凝胶/壳聚糖复合药物载体材料,采用扫描电镜(SEM)、红外光谱(F TIR)等对复合材料的结构形貌进行了分析,研究了复合材料对硫酸庆大霉素药物的担载和释放性能。
结果表明,所得SiO2气凝胶/壳聚糖复合材料为多孔网络结构,其中,由450℃处理的SiO2气凝胶制得的气凝胶/壳聚糖复合材料对硫酸庆大霉素具有较好的药物担载和缓释性能。
关键词: SiO2气凝胶;壳聚糖;复合材料;多孔;药物载体中图分类号: TB382文献标识码:A 文章编号:100129731(2007)09215272041 引 言多孔材料由于具有高比表面积及孔径结构可调等特性,作为药物载体,既可达到较高的载药量,又可获得理想的药物缓释效果,因而多孔材料作为药物载体有着其独特的优势。
目前,多孔生物陶瓷[1]和多孔微晶玻璃[2]等作为药物载体材料的研究已有报道。
SiO2气凝胶是一种无毒的多孔无机材料,具有超低密度、大孔隙率、高比表面积和良好的生物相容性等特殊性质。
近几年,SiO2气凝胶和干凝胶作为药物载体和缓释材料的研究开始受到人们的关注[3~5]。
壳聚糖(CS)是甲壳素脱乙酰化产物,其基本结构单位为葡萄糖胺,具有良好的生物相容性、生物降解性和杀菌作用。
目前,壳聚糖作为药物载体的研究已有较多报道,研究主要体现在壳聚糖载药微球[6]和壳聚糖复合材料药物载体[7]方面。
据报道[8,9],多孔的SiO2/壳聚糖凝胶能够成功用于葡萄糖氧化酶的固定;而利用溶胶2凝胶法和超临界干燥工艺制备的壳聚糖/SiO2复合气凝胶具有较小的细胞毒性和较好的生物相容性,有望用于药物载体和废水处理等领域。
微波辅助加热法制备晶种用于高浓度硫酸氧钛溶液水解制钛白研究
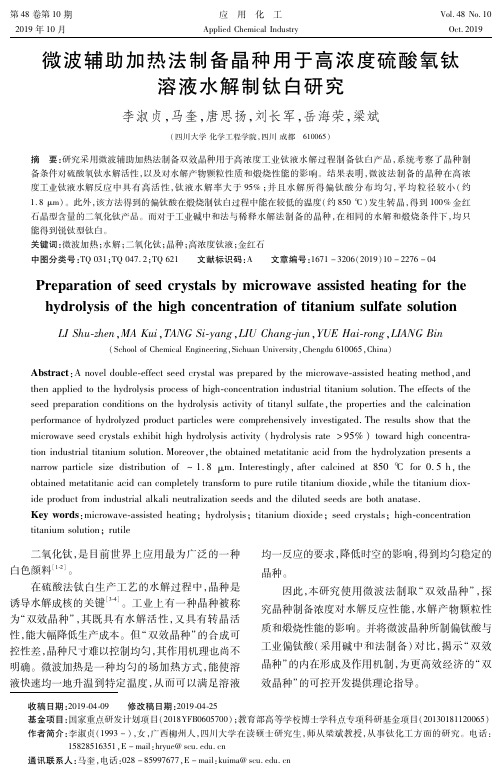
均一反应的要求,降低时空的影响,得到均匀稳定的 晶种。
因此,本研 究 使 用 微 波 法 制 取 “双 效 晶 种 ”,探 究晶种制备浓度对水解反应性能,水解产物颗粒性 质和煅烧性能的影响。并将微波晶种所制偏钛酸与 工业偏钛酸(采 用 碱 中 和 法 制 备 )对 比,揭 示 “双 效 晶种”的内在形成及作用机制,为更高效经济的“双 效晶种”的可控开发提供理论指导。
Preparationofseedcrystalsbymicrowaveassistedheatingforthe hydrolysisofthehighconcentrationoftitanium sulfatesolution
LIShuzhen,MAKui,TANGSiyang,LIUChangjun,YUEHairong,LIANGBin
收稿日期:20190409 修改稿日期:20190425 基金项目:国家重点研发计划项目(2018YFB0605700);教育部高等学校博士学科点专项科研基金项目(20130181120065) 作者简介:李淑贞(1993-),女,广西柳州人,四川大学在读硕士研究生,师从梁斌教授,从事钛化工方面的研究。电话:
经过酸解、沉降过滤、浓缩等处理后所得,各项指标 如下:钛 含 量 (以 TiO2 计)为 215g/L,铁 钛 比 为 0.25,F值 (钛 液 中 有 效 酸 与 二 氧 化 钛 质 量 比)为 192,三价钛含量为 0.55g/L,稳定性是 550。 1.2 实验方法
(SchoolofChemicalEngineering,SichuanUniversity,Chengdu610065,China)
Abstract:Anoveldoubleeffectseedcrystalwaspreparedbythemicrowaveassistedheatingmethod,and thenappliedtothehydrolysisprocessofhighconcentrationindustrialtitaniumsolution.Theeffectsofthe seedpreparationconditionsonthehydrolysisactivityoftitanylsulfate,thepropertiesandthecalcination performanceofhydrolyzedproductparticleswerecomprehensivelyinvestigated.Theresultsshowthatthe microwaveseedcrystalsexhibithighhydrolysisactivity(hydrolysisrate>95%)towardhighconcentra tionindustrialtitanium solution.Moreover,theobtainedmetatitanicacidfrom thehydrolyzationpresentsa narrowparticlesizedistributionof~1.8μm.Interestingly,aftercalcinedat850℃ for0.5h,the obtainedmetatitanicacidcancompletelytransformtopurerutiletitaniumdioxide,whilethetitaniumdiox ideproductfrom industrialalkalineutralizationseedsandthedilutedseedsarebothanatase. Keywords:microwaveassistedheating;hydrolysis;titanium dioxide;seedcrystals;highconcentration titanium solution;rutile
液-液溶剂置换法制备超疏水SiO2气凝胶
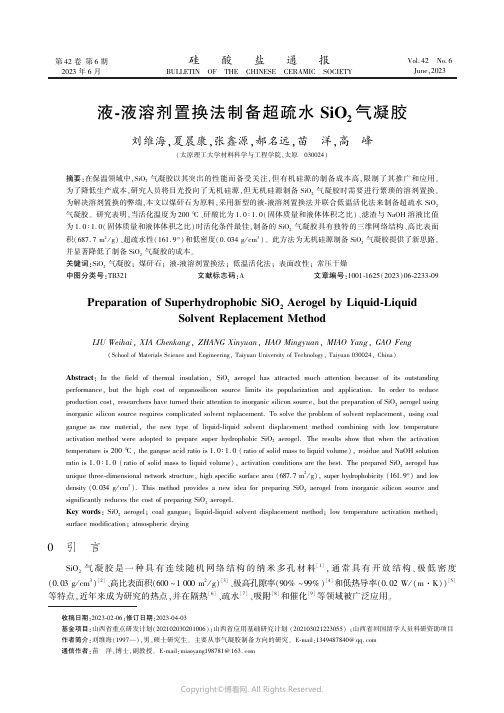
第42卷第6期2023年6月硅㊀酸㊀盐㊀通㊀报BULLETIN OF THE CHINESE CERAMIC SOCIETY Vol.42㊀No.6June,2023液-液溶剂置换法制备超疏水SiO 2气凝胶刘维海,夏晨康,张鑫源,郝名远,苗㊀洋,高㊀峰(太原理工大学材料科学与工程学院,太原㊀030024)摘要:在保温领域中,SiO 2气凝胶以其突出的性能而备受关注,但有机硅源的制备成本高,限制了其推广和应用㊂为了降低生产成本,研究人员将目光投向了无机硅源,但无机硅源制备SiO 2气凝胶时需要进行繁琐的溶剂置换㊂为解决溶剂置换的弊端,本文以煤矸石为原料,采用新型的液-液溶剂置换法并联合低温活化法来制备超疏水SiO 2气凝胶㊂研究表明,当活化温度为200ħ㊁矸酸比为1.0ʒ1.0(固体质量和液体体积之比)㊁滤渣与NaOH 溶液比值为1.0ʒ1.0(固体质量和液体体积之比)时活化条件最佳,制备的SiO 2气凝胶具有独特的三维网络结构㊁高比表面积(687.7m 2/g)㊁超疏水性(161.9ʎ)和低密度(0.034g /cm 3)㊂此方法为无机硅源制备SiO 2气凝胶提供了新思路,并显著降低了制备SiO 2气凝胶的成本㊂关键词:SiO 2气凝胶;煤矸石;液-液溶剂置换法;低温活化法;表面改性;常压干燥中图分类号:TB321㊀㊀文献标志码:A ㊀㊀文章编号:1001-1625(2023)06-2233-09Preparation of Superhydrophobic SiO 2Aerogel by Liquid-Liquid Solvent Replacement MethodLIU Weihai ,XIA Chenkang ,ZHANG Xinyuan ,HAO Mingyuan ,MIAO Yang ,GAO Feng (School of Materials Science and Engineering,Taiyuan University of Technology,Taiyuan 030024,China)Abstract :In the field of thermal insulation,SiO 2aerogel has attracted much attention because of its outstanding performance,but the high cost of organosilicon source limits its popularization and application.In order to reduce production cost,researchers have turned their attention to inorganic silicon source,but the preparation of SiO 2aerogel using inorganic silicon source requires complicated solvent replacement.To solve the problem of solvent replacement,using coal gangue as raw material,the new type of liquid-liquid solvent displacement method combining with low temperature activation method were adopted to prepare super hydrophobic SiO 2aerogel.The results show that when the activation temperature is 200ħ,the gangue acid ratio is 1.0ʒ1.0(ratio of solid mass to liquid volume),residue and NaOH solution ratio is 1.0ʒ1.0(ratio of solid mass to liquid volume),activation conditions are the best.The prepared SiO 2aerogel has unique three-dimensional network structure,high specific surface area (687.7m 2/g),super hydrophobicity (161.9ʎ)and low density (0.034g/cm 3).This method provides a new idea for preparing SiO 2aerogel from inorganic silicon source and significantly reduces the cost of preparing SiO 2aerogel.Key words :SiO 2aerogel;coal gangue;liquid-liquid solvent displacement method;low temperature activation method;surface modification;atmospheric drying 收稿日期:2023-02-06;修订日期:2023-04-03基金项目:山西省重点研发计划(202102030201006);山西省应用基础研究计划(202103021223055);山西省回国留学人员科研资助项目作者简介:刘维海(1997 ),男,硕士研究生㊂主要从事气凝胶制备方向的研究㊂E-mail:1349487840@通信作者:苗㊀洋,博士,副教授㊂E-mail:miaoyang198781@ 0㊀引㊀言SiO 2气凝胶是一种具有连续随机网络结构的纳米多孔材料[1],通常具有开放结构㊁极低密度(0.03g /cm 3)[2]㊁高比表面积(600~1000m 2/g)[3]㊁极高孔隙率(90%~99%)[4]和低热导率(0.02W/(m㊃K))[5]等特点,近年来成为研究的热点,并在隔热[6]㊁疏水[7]㊁吸附[8]和催化[9]等领域被广泛应用㊂2234㊀新型功能材料硅酸盐通报㊀㊀㊀㊀㊀㊀第42卷近年来,由于有机硅源制备气凝胶的成本较高,利用固废材料制备气凝胶引起了人们的关注,其中煤矸石含硅量高,被认为是一种新的生产原料,而且采用煤矸石制备的SiO 2气凝胶中含有少量Al 2O 3,因此比纯SiO 2气凝胶具有更高的热稳定性,更适用于各种应用场景[10]㊂煤矸石的高附加值利用主要依赖于其活化程度和硅铝分离,而目前煤矸石的活化主要采用两种方法:一种是将煤矸石置于650ħ下煅烧,使高岭石变为无定形的SiO 2和Al 2O 3,但浸出率低[11];另一种是将煤矸石与碳酸钠混合均匀,放入马弗炉中于815ħ下煅烧,形成易溶解的霞石[12]㊂这两种方法不仅需要在较高温度下活化,而且硅铝分离不彻底,容易造成二次污染㊂本文采用低温活化法,在提供相同活化效果的同时,不仅将传统活化温度从800ħ降低到200ħ,还可以得到无定形的SiO 2和纯度为97.29%(质量分数)的硫酸铝,且不产生二次废物㊂以往采用无机硅源作为制备气凝胶的前驱体来降低成本,但存在两个问题:一是以硅酸钠为前驱体制备SiO 2气凝胶的过程中涉及到Na +的脱除,该过程是一个非常重要但成本高昂的步骤[13];二是湿凝胶需进行繁琐的有机溶剂置换,这会消耗大量有机溶剂,并且造成二次环境破坏[5]㊂本研究提出的液-液溶剂置换法可以直接置换湿凝胶体系中的水溶液和NaCl,使制备的气凝胶中Na +含量极低㊂因此,研究证明液-液溶剂置换法和低温活化法是提高气凝胶大规模生产和降低成本的简单可行方法㊂1㊀实㊀验1.1㊀原材料试验所用的原材料有煤矸石㊁正己烷㊁三甲基氯硅烷(TMCS)㊁H 2SO 4(ȡ98%,质量分数)㊁NaOH㊁HCl 和去离子水,化学试剂均为分析纯㊂煤矸石的主要化学组成如表1所示㊂图1为煤矸石的XRD 谱,由图1可知,煤矸石主要矿物成分为高岭石㊂表1㊀煤矸石的主要化学组成Table 1㊀Main chemical composition of coal gangueComposition SiO 2aerogel Al 2O 3TiO 2Na 2O Fe 2O 3SO 2Other LOI Mass fraction /%44.4438.400.820.250.240 1.4514.4图1㊀煤矸石的XRD 谱Fig.1㊀XRD pattern of coal gangue1.2㊀制备过程以煤矸石为原料制备SiO 2气凝胶的流程图如图2所示㊂该工艺分为四个步骤:煤矸石的预处理㊁湿凝胶的合成㊁液-液溶剂置换㊁气凝胶的表面改性和干燥㊂煤矸石的预处理:称取10g 煤矸石,并与不同体积的H 2SO 4(ȡ98%,质量分数)混合,得到不同比例的煤矸石与H 2SO 4(ȡ98%)的混合物,矸酸比(固体质量与液体的体积比)依次为1.0ʒ0.8㊁1.0ʒ0.9㊁1.0ʒ1.0㊁1.0ʒ1.1㊁1.0ʒ1.2㊂然后将混合物在不同温度(50㊁100㊁150㊁200㊁250ħ)的油浴锅中活化2h,混合物在锅内冷却至室温㊂湿凝胶的制备:将活化后的混合物和一定量的去离子水混合搅拌后过滤,滤渣洗涤干燥㊂将滤渣和浓度为2mol /L 的NaOH 溶液混合,滤渣和NaOH 溶液的固液比(固体质量与液体的体积比)依次为0.8ʒ1.0㊁0.9ʒ1.0㊁1.0ʒ1.0㊁1.1ʒ1.0㊁1.2ʒ1.0,在该条件下制备的气凝胶依次命名为CGBA-1,CGBA-2,CGBA-3,CGBA-4,CGBA-5,然后将混合物在80ħ的水浴锅中搅拌4h,混合物在锅内冷却到室温,真空过滤得到硅溶胶㊂取上述获得的硅溶胶,加入3mol /L 的盐酸调节pH 值,静置1d㊂硅溶胶通过缓慢溶胶㊁凝胶变为湿凝胶,常温老化2d 后再通过超声波分散得到小的湿凝胶颗粒㊂液-液溶剂置换:将凝胶颗粒完全浸没在正己烷中,并将其悬浮在正己烷溶液2/3高度处,密封在玻璃瓶内,置于50ħ的水浴锅中,保温3d㊂第6期刘维海等:液-液溶剂置换法制备超疏水SiO 2气凝胶2235㊀图2㊀以煤矸石为原料制备SiO 2气凝胶的流程Fig.2㊀Process of preparing SiO 2aerogel with coal gangue as raw material 气凝胶的表面改性和干燥:将完成置换的凝胶颗粒浸入TMCS 和正己烷溶液的混合溶液中进行改性,改性成功后用正己烷清洗,抽滤后依次置于60和120ħ下干燥2h,得到SiO 2气凝胶㊂1.3㊀样品表征采用TD-3500型X 射线衍射仪对SiO 2气凝胶和中间产物进行物相表征,采用Gemimi 300型扫描电子显微镜观察SiO 2气凝胶和中间产物的微观形貌,采用JW-BK122W 型比表面及孔径吸附仪对样品的比表面积及孔径分布进行测试,采用ALPHA Ⅱ型傅里叶变换红外光谱仪对SiO 2气凝胶的化学键与官能团进行分析,采用JC2000D1型接触角测量仪对SiO 2气凝胶的疏水性能进行测试㊂2㊀结果与讨论2.1㊀不同活化温度与矸酸比对煤矸石活化的影响图3为煤矸石在不同条件下铝的溶出率及中间产物的XRD 谱㊂考察矸酸比及活化温度对铝溶出率的影响后,可以确定矸酸比的最佳比值为1.0ʒ1.0,最佳活化温度为200ħ,此时铝溶出率达到85.9%,如图3(a)所示㊂由图3(b)可知,煤矸石与H 2SO 4(ȡ98%)的活化混合物在不同温度(150㊁200和250ħ)活化后存在两种类型的反应产物:水合硫酸铝((H 3O)(Al(SO 4)2))和硫酸铝(Al 2(SO 4)3),表明在150ħ活化温度下,煤矸石中的高岭石可以与H 2SO 4(ȡ98%)反应生成水合硫酸铝,当温度高于200ħ时,反应生成硫酸铝㊂煤矸石和250ħ活化产物的SEM 照片分别如图4(a)㊁(b)所示,活化前煤矸石是六边形鳞片状的高岭石,而活化后主要为硫酸铝的方块和无定形SiO 2㊂对滤渣进行物相分析,在50和100ħ的活化温度下,滤渣与活化前煤矸石的主要矿物成分基本相同,均属于高岭石,表明该温度下煤矸石未被活化(见图4(a))㊂在较低的活化温度下,铝的溶出率小于10%,只有一部分高活性的Al 2O 3与H 2SO 4(ȡ98%)发生反应㊂在高于150ħ的情况下,滤渣主要由无定形产物组成㊂如图4(c)所示,滤渣的SEM 照片表明,硫酸铝已完全溶解在去离子水中㊂从图4(c)可以看出,滤渣大部分为不规则形状固体㊂如图4(d)所示,无定形产物主要组成元素是硅和氧㊂表2为不同中间产物及SiO 2气凝胶的主要化学组成㊂从表2中可知,SiO 2含量达到74.74%,Al 2O 3仅为8.16%㊂滤液干燥得到的晶体XRD 谱表明(见图3(c)),其主要成分为水合硫酸铝和硫酸铝,且从表2可知,硫酸铝(SO 2+Al 2O 3)的纯度达到97.29%㊂高岭石与H 2SO 4(ȡ98%)的理想化学反应式如式(1)~(3)所示,其中式(2)的活化温度为150ħ,式(3)活化温度为200ħ及以上㊂2236㊀新型功能材料硅酸盐通报㊀㊀㊀㊀㊀㊀第42卷图3㊀煤矸石在不同条件下铝的溶出率及中间产物的XRD谱Fig.3㊀Aluminum dissolution rate of coal gangue under different conditions and XRD patterns of intermediateproduct第6期刘维海等:液-液溶剂置换法制备超疏水SiO 2气凝胶2237㊀图4㊀煤矸石㊁活化煤矸石和煤矸石滤渣的SEM 照片和煤矸石滤渣的EDS 照片Fig.4㊀SEM images of coal gangue,activated coal gangue and coal gangue filter residue and EDS images of coal gangue filter residue 2SiO 2㊃Al 2O 3㊃2H 2O 2SiO 2㊃Al 2O 3+2H 2O (1)SiO 2㊃Al 2O 3+4H 2SO 4 SiO 2+2(H 3O)(Al(SO 4)2)+H 2O (2)2SiO 2㊃Al 2O 3+3H 2SO 4 2SiO 2+Al 2(SO 4)3+3H 2O (3)根据式(2)和(3),足量的H 2SO 4(ȡ98%)和温度是获得反应产物SiO 2和Al 2(SO 4)3的关键因素㊂但是,当使用过量的H 2SO 4(ȡ98%)时,H 2SO 4作为杂质存在于混合物中,得到的滤水溶液PH 值过低,不利于Al 2(SO 4)3回收㊂图3(a)中矸酸比为1.0ʒ1.0,随着活化温度从150ħ上升到200ħ,铝的溶出率明显增加㊂根据式(1)~(3),在200ħ时活化相同质量的SiO 2㊃Al 2O 3㊃2H 2O 比在150ħ时少用1/4的H 2SO 4(ȡ98%)㊂经综合考虑活化温度为200ħ为最佳㊂表2㊀不同中间产物及SiO 2气凝胶的主要化学组成Table 2㊀Main chemical composition of different intermediate products and SiO 2aerogel Type Mass fraction /%SiO 2Al 2O 3TiO 2Na 2O Fe 2O 3SO 3Other LOI Filter residue 74.748.160.2800.100.33 1.6714.74Filtrate 026.530.9200.4570.76 1.340SiO 2aerogel 81.920.920.010.200.07 1.5715.312.2㊀液-液溶剂置换法对气凝胶的影响液-液溶剂置换原理:将湿凝胶置于正己烷中一段时间,少量水溶液与正己烷互溶后呈互溶饱和状态,并保持动态平衡㊂将湿凝胶悬浮在正己烷中,动态平衡被打破㊂由于凝胶中的水比正己烷密度大,如果不被凝胶骨架的束缚,它就会自动向势能低的地方流动㊂因此,凝胶中的水溶液倾向于熵增㊂液-液溶剂置换模型和水溶液干燥晶体的XRD 谱如图5所示,在液-液界面,水和正己烷的交换率不同,在凝胶的液-液界面,总趋势是H 2O㊁Cl -和Na +扩散到正己烷中,而在底部溶液的液-液界面,却是H 2O㊁Cl -和Na +从正己烷扩散到水溶液中㊂随着时间的推移,越来越多的水溶液聚集在底部,直到凝胶中的大部分水溶液被置换出来㊂如图5所示,置换后的水溶液中含有大量NaCl㊂通常无机硅源不经过特殊处理时制备的气凝胶中会含有较多Na +,而Na +通过碱性溶解和阻断介孔来减少孔体积,使气凝胶的高温性能急剧下降[13]㊂图6为CGBA-3的EDS 照片㊂从表2和图6可以看出,本研究制备的SiO 2气凝胶基本不含Na +,不会对SiO 2气凝胶产生影响,这说明液-液溶剂置换法可以置换出大部分的NaCl㊂与传统的溶剂置换法相比,液-液溶剂置换法直接省去了乙醇置换和正己烷置换,溶剂用量减少了95%,且用过的溶剂可以重复使用㊂该方法在溶剂置换过程中能直接去除Na +,省去昂贵的Na +去除过程㊂因此,液-液溶剂置换模型是可行的,该过程不会破坏凝胶骨架,骨架结构将得到完整保存㊂2238㊀新型功能材料硅酸盐通报㊀㊀㊀㊀㊀㊀第42卷图5㊀液-液溶剂置换模型和水溶液干燥晶体的XRD 谱Fig.5㊀Liquid-liquid solvent displacement model and XRD pattern of dried crystals in aqueoussolution 图6㊀CGBA-3的EDS 照片Fig.6㊀EDS images of CGBA-32.3㊀气凝胶的表征图7为滤渣于NaOH 溶液中固液相的不同配比对样品微观结构和性能的影响㊂图7(a)为不同矸酸比对SiO 2浸出率的影响,从图7(a)中可以看出,随着矸酸比的增加,浸出率逐渐上升,当矸酸比达到1.0ʒ1.0时,浸出率达到最大,此时浸出率为86.25%㊂图7(b)为SiO 2气凝胶的FTIR 光谱,Si O Si 不对称㊁对称和摇摆弯曲振动的峰值分别在1083㊁795㊁471cm -1左右[14];在1634㊁3444cm -1处的峰对应于O H [12];在955cm -1附㊀第6期刘维海等:液-液溶剂置换法制备超疏水SiO2气凝胶2239近观察到的吸收带是由于Si C键的拉伸振动,而在1398cm-1处的吸收带对应Si C键和C H键[15]㊂Si C键和C H键的存在表明表面修饰成功,这是疏水性的主要来源㊂图8为CGBA-1~CGBA-5的SEM 照片和疏水角照片,如图8所示,疏水角最高可达161.9ʎ,比刘维海等[10]制备的气凝胶疏水角高10ʎ㊂如图7(c)所示,从样品的N2吸附-脱附曲线可知,所有样品的回线与H4型滞后环相对应,说明制备的SiO2气凝胶是典型的介孔材料;图7(d)为样品的孔径分布图,可以看出气凝胶孔径分布比较集中㊂固液比越小,孔径分布越集中㊂从图7(e)中可以看出,随着固液比的增加,气凝胶的比表面积先增大后减小㊂当固液比为1.0ʒ1.0时达到最大值㊂结合如图8所示的样品表观形貌可以确认制备的SiO2气凝胶具有均匀的骨架结构㊂当固液比低时,凝胶前驱体浓度过高,凝胶形成过程中颗粒间的团簇使最终构成骨架结构的颗粒变粗,虽更有利于抵抗表面张力,骨架坍塌的较小,但使样品致密,比表面积变小㊂随着固液比的增加,孔径分布变得更加分散,平均孔径变小;当固液比高时,凝胶前驱体浓度过低,颗粒更小,凝胶过程中颗粒间团簇构成了最终的骨架结构,随着老化,骨架颗粒的脖颈长不好,常压干燥时脖颈处最先断裂造成部分坍塌㊂当固液比为1.0ʒ1.0时,骨架结构较粗,颗粒间的脖颈得到有效增长,干燥时不易断裂收缩,比表面积高达687.7m2/g,且测得气凝胶的密度为0.034g/cm3㊂因此固液比取1.0ʒ1.0为最佳㊂为了研究气凝胶的热稳定性,使用TG-DSC进行了研究㊂图7(f)是气凝胶在10ħ/min升温速率下的TG曲线㊂在空气气氛下,随着温度的升高,气凝胶的质量不断减小㊂从0~380ħ,质量损失约为3%,这是由湿凝胶干燥阶段未完全脱附的有机溶剂蒸发导致的㊂在380~431ħ时失重迅速(约4%),这是附着在气凝胶表面的 CH3大量燃烧所致㊂当温度在380ħ左右时,气凝胶表面仍有大量的 CH3存在,因此SiO2气凝胶仍具有疏水性㊂从431~900ħ的质量损失趋势减弱,此时气凝胶只剩下少量残留物,继续挥发㊂总质量损失在12%~16%㊂图8为CGBA-1~CGBA-5的SEM照片和疏水角照片㊂从图8中可以看出,SiO2气凝胶具有独特的三维网络结构,由十几到几十纳米的小颗粒相互连接形成珍珠链状结构,这些链状物相互交错构成了SiO2气凝胶的骨架,使得SiO2气凝胶整体结构呈多孔状网络结构㊂随着硅酸浓度的增加,链状结构逐渐变粗,介孔变得更加均匀㊂当硅酸浓度低时,SiO2气凝胶的骨架较细,导致干燥时骨架被破坏,形成大孔,使介孔不均匀㊂2240㊀新型功能材料硅酸盐通报㊀㊀㊀㊀㊀㊀第42卷图7㊀CGBA-3的物理性能Fig.7㊀Physical properties ofCGBA-3图8㊀CGBA-1~CGBA-5的SEM 照片和疏水角照片Fig.8㊀SEM images and hydrophobic angle images of CGBA-1~CGBA-53㊀结㊀论1)在煤矸石的低温活化阶段,产物随活化温度的变化而变化㊂煤矸石制备气凝胶试验表明,活化温度为200ħ㊁矸酸比为1.0ʒ1.0㊁滤渣与NaOH 的固液比为1.0ʒ1.0时活化条件最佳㊂在提供相同活化效果的情况下,低温活化法不仅将传统活化温度从800ħ降低到200ħ,而且可以获得无定形SiO 2和纯度为97.29%(质量分数)的硫酸铝,且无二次废物产生㊂2)在湿凝胶溶剂置换阶段引入了液-液溶剂置换法,与传统溶剂置换方法相比,液-液溶剂置换法将繁琐的乙醇置换和正己烷置换合并为一步置换,减少了95%的溶剂用量,且溶剂可直接重复使用㊂该方法在溶剂置换过程中可直接去除Na +,省去具有昂贵成本的Na +去除过程,大大降低了制备成本㊂3)SiO 2气凝胶的晶型为无定形,微观呈多孔三维网络结构,具有高比表面积(687.7m 2/g)㊁超疏水性(接触角为161.9ʎ)和低堆积密度(0.034g /cm 3)的优异性能㊂㊀第6期刘维海等:液-液溶剂置换法制备超疏水SiO2气凝胶2241参考文献[1]㊀ZHU J,GUO S,LI X.Preparation of hydrophobic silica aerogel with kaolin dried at ambient pressure.RSC Advances,2015,5(125):103656-103661.[2]㊀HU W B,LI M M,CHEN W,et al.Preparation of hydrophobic silica aerogel with Kaolin dried at ambient pressure[J].Colloids and SurfacesA:Physicochemical and Engineering Aspects,2016,501:83-91.[3]㊀徐㊀静,赵永奇,徐成强,等.硅酸钠模数对SiO2气凝胶结构和表面改性机制的影响[J].硅酸盐通报,2022,41(10):3667-3674.XU J,ZHAO Y Q,XU C Q,et al.Effect of sodium silicate modulus on structure and surface modification mechanism of SiO2aerogel[J].Bulletin of the Chinese Ceramic Society,2022,41(10):3667-3674(in Chinese).[4]㊀KANTOR Z,WU T T,ZENG Z H,et al.Heterogeneous silica-polyimide aerogel-in-aerogel nanocomposites[J].Chemical Engineering Journal,2022,443:136401.[5]㊀KHEDKAR M V,SOMVANSHI S B,HUMBE A V,et al.Surface modified sodium silicate based superhydrophobic silica aerogels prepared viaambient pressure drying process[J].Journal of Non-Crystalline Solids,2019,511:140-146.[6]㊀何㊀辉,姜勇刚,张忠明,等.二氧化硅气凝胶隔热复合材料的高温疏水改性及失效机制[J].硅酸盐通报,2022,41(5):1813-1820.HE H,JIANG Y G,ZHANG Z M,et al.High Temperature hydrophobic modification and failure mechanism of silica aerogel thermal insulation composites[J].Bulletin of the Chinese Ceramic Society,2022,41(5):1813-1820(in Chinese).[7]㊀夏晨康,李淑敏,胡㊀博,等.以煤矸石为原料制备Al2O3-SiO2气凝胶的工艺与性能[J].硅酸盐通报,2022,41(2):607-615.XIA C K,LI S M,HU B,et al.Preparation and properties of Al2O3-SiO2aerogel from coal gangue[J].Bulletin of the Chinese Ceramic Society, 2022,41(2):607-615(in Chinese).[8]㊀DIAO S,LIU H E,CHEN S,et al.Oil adsorption performance of graphene aerogels[J].Journal of Materials Science,2020,55(11):4578-4591.[9]㊀董龙浩,韩㊀磊,田㊀亮,等.TiO2气凝胶的制备及光催化性能研究进展[J].硅酸盐通报,2020,39(1):290-302.DONG L H,HAN L,TIAN L,et al.Research progress of preparation and photocatalytic performance of TiO2aerogel[J].Bulletin of the Chinese Ceramic Society,2020,39(1):290-302.[10]㊀刘维海,李淑敏,武泽民,等.煤矸石酸碱一步催化法制备SiO2气凝胶[J].矿产保护与利用,2022,42(1):165-171.LIU W H,LI S M,WU Z M,et al.Preparation of SiO2aerogel from coal gangue by one-step acid-base catalytic method[J].Conservation and Utilization of Mineral Resources,2022,42(1):165-171(in Chinese).[11]㊀ZHU X D,CHEN S,CHEN B W,et al.Thermal treatment of coal-gangue activation and influential factors[J].Advanced Materials Research,2013,750/751/752:1309-1312.[12]㊀WEI J,ZHU P H,SUN H.Ambient-dried silica aerogel powders derived from coal gangue by using one-pot method[J].Materials(Basel,Switzerland),2022,15(4):1454.[13]㊀LUO Z T,YANG G J,ZHANG M,et al.Effect of residual Na+on the properties of aerogel prepared with sodium silicate via APD[J].Journalof Non-Crystalline Solids,2022,575:121200.[14]㊀SHEN M M,JIANG X Y,ZHANG M,et al.Synthesis of SiO2-Al2O3composite aerogel from fly ash:a low-cost and facile approach[J].Journalof Sol-Gel Science and Technology,2020,93(2):281-290.[15]㊀李贵安,朱庭良,叶录元,等.原位法常压干燥制备疏水SiO2气凝胶及其热稳定性[J].物理化学学报,2009,25(9):1811-1815.LI G A,ZHU T L,YE L Y,et al.Hydrophobic silica aerogel prepared in-situ by ambient pressure drying and its thermal stability[J].Acta Physico-Chimica Sinica,2009,25(9):1811-1815(in Chinese).。
PREPARATION OF HYDROGEL
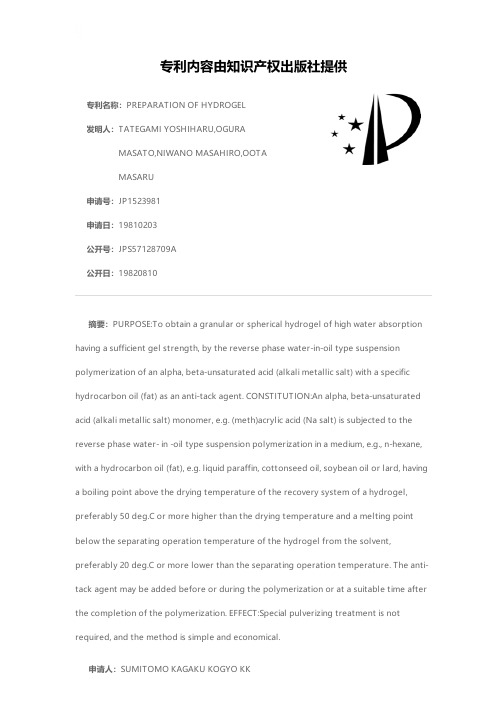
专利名称:PREPARATION OF HYDROGEL发明人:TATEGAMI YOSHIHARU,OGURAMASATO,NIWANO MASAHIRO,OOTAMASARU申请号:JP1523981申请日:19810203公开号:JPS57128709A公开日:19820810专利内容由知识产权出版社提供摘要:PURPOSE:To obtain a granular or spherical hydrogel of high water absorption having a sufficient gel strength, by the reverse phase water-in-oil type suspension polymerization of an alpha, beta-unsaturated acid (alkali metallic salt) with a specific hydrocarbon oil (fat) as an anti-tack agent. CONSTITUTION:An alpha, beta-unsaturated acid (alkali metallic salt) monomer, e.g. (meth)acrylic acid (Na salt) is subjected to the reverse phase water- in -oil type suspension polymerization in a medium, e.g., n-hexane, with a hydrocarbon oil (fat), e.g. liquid paraffin, cottonseed oil, soybean oil or lard, having a boiling point above the drying temperature of the recovery system of a hydrogel, preferably 50 deg.C or more higher than the drying temperature and a melting point below the separating operation temperature of the hydrogel from the solvent, preferably 20 deg.C or more lower than the separating operation temperature. The anti-tack agent may be added before or during the polymerization or at a suitable time after the completion of the polymerization. EFFECT:Special pulverizing treatment is not required, and the method is simple and economical.申请人:SUMITOMO KAGAKU KOGYO KK更多信息请下载全文后查看。
Preparation of hydrophobic organic aeorgels
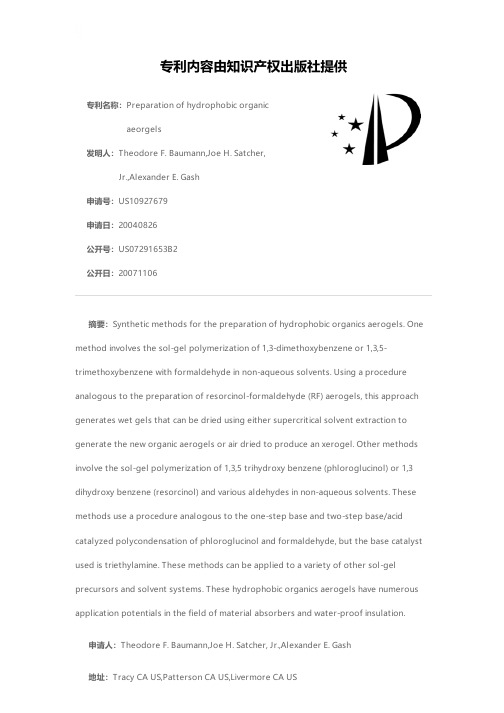
专利名称:Preparation of hydrophobic organic aeorgels发明人:Theodore F. Baumann,Joe H. Satcher, Jr.,Alexander E. Gash申请号:US10927679申请日:20040826公开号:US07291653B2公开日:20071106专利内容由知识产权出版社提供摘要:Synthetic methods for the preparation of hydrophobic organics aerogels. One method involves the sol-gel polymerization of 1,3-dimethoxybenzene or 1,3,5-trimethoxybenzene with formaldehyde in non-aqueous solvents. Using a procedure analogous to the preparation of resorcinol-formaldehyde (RF) aerogels, this approach generates wet gels that can be dried using either supercritical solvent extraction to generate the new organic aerogels or air dried to produce an xerogel. Other methods involve the sol-gel polymerization of 1,3,5 trihydroxy benzene (phloroglucinol) or 1,3 dihydroxy benzene (resorcinol) and various aldehydes in non-aqueous solvents. These methods use a procedure analogous to the one-step base and two-step base/acid catalyzed polycondensation of phloroglucinol and formaldehyde, but the base catalyst used is triethylamine. These methods can be applied to a variety of other sol-gel precursors and solvent systems. These hydrophobic organics aerogels have numerous application potentials in the field of material absorbers and water-proof insulation.申请人:Theodore F. Baumann,Joe H. Satcher, Jr.,Alexander E. Gash地址:Tracy CA US,Patterson CA US,Livermore CA US国籍:US,US,US代理人:Ann M. Lee,Alan H. Thompson,John H. Lee 更多信息请下载全文后查看。
高分子材料纳米二氧化硅外文文献翻译
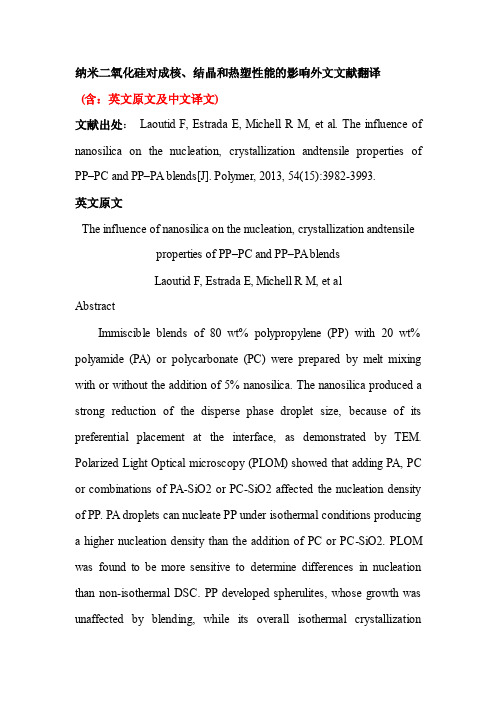
纳米二氧化硅对成核、结晶和热塑性能的影响外文文献翻译(含:英文原文及中文译文)文献出处:Laoutid F, Estrada E, Michell R M, et al. The influence of nanosilica on the nucleation, crystallization andtensile properties of PP–PC and PP–PA blends[J]. Polymer, 2013, 54(15):3982-3993.英文原文The influence of nanosilica on the nucleation, crystallization andtensileproperties of PP–PC and PP–PA blendsLaoutid F, Estrada E, Michell R M, et alAbstractImmiscible blends of 80 wt% polypropylene (PP) with 20 wt% polyamide (PA) or polycarbonate (PC) were prepared by melt mixing with or without the addition of 5% nanosilica. The nanosilica produced a strong reduction of the disperse phase droplet size, because of its preferential placement at the interface, as demonstrated by TEM. Polarized Light Optical microscopy (PLOM) showed that adding PA, PC or combinations of PA-SiO2 or PC-SiO2 affected the nucleation density of PP. PA droplets can nucleate PP under isothermal conditions producing a higher nucleation density than the addition of PC or PC-SiO2. PLOM was found to be more sensitive to determine differences in nucleation than non-isothermal DSC. PP developed spherulites, whose growth was unaffected by blending, while its overall isothermal crystallizationkinetics was strongly influenced by nucleation effects caused by blending. Addition of nanosilica resulted in an enhancement of the strain at break of PP-PC blends whereas it was observed to weaken PP-PA blends. Keywords:Nanosilica,Nucleation,PP blends1 OverviewImmiscible polymer blends have attracted attention for decades because of their potential application as a simple route to tailor polymer properties. The tension is in two immiscible polymerization stages. This effect usually produces a transfer phase between the pressures that may allow the size of the dispersed phase to be allowed, leading to improved mixing performance.Block copolymers and graft copolymers, as well as some functional polymers. For example, maleic anhydride grafted polyolefins act as compatibilizers in both chemical affinities. They can reduce the droplet volume at the interface by preventing the two polymers from coalescing. In recent years, various studies have emphasized that nanofillers, such as clay carbon nanotubes and silica, can be used as a substitute for organic solubilizers for incompatible polymer morphology-stabilized blends. In addition, in some cases, nanoparticles in combination with other solubilizers promote nanoparticle interface position.The use of solid particle-stabilized emulsions was first discovered in 1907 by Pickering in the case of oil/emulsion containing colloidalparticles. In the production of so-called "Pickling emulsions", solid nanoparticles can be trapped in the interfacial tension between the two immiscible liquids.Some studies have attempted to infer the results of blending with colloidal emulsion polymer blends. Wellman et al. showed that nanosilica particles can be used to inhibit coalescence in poly(dimethylsiloxane)/polyisobutylene polymers. mix. Elias et al. reported that high-temperature silicon nanoparticles can migrate under certain conditions. The polypropylene/polystyrene and PP/polyvinyl acetate blend interfaces form a mechanical barrier to prevent coalescence and reduce the size of the disperse phase.In contrast to the above copolymers and functionalized polymers, the nanoparticles are stable at the interface due to their dual chemical nature. For example, silica can affect nanoparticle-polymer affinities locally, minimizing the total free energy that develops toward the system.The nanofiller is preferentially placed in equilibrium and the wetting parameters can be predicted and calculated. The difference in the interfacial tension between the polymer and the nanoparticles depends on the situation. The free-diffusion of the nanoparticle, which induces the nanoparticles and the dispersed polymer, occurs during the high shear process and shows that the limitation of the viscosity of the polymer hardly affects the Brownian motion.As a result, nanoparticles will exhibit strong affinity at the local interface due to viscosity and diffusion issues. Block copolymers need to chemically target a particular polymer to the nanoparticle may provide a "more generic" way to stabilize the two-phase system.Incorporation of nanosilica may also affect the performance of other blends. To improve the distribution and dispersion of the second stage, mixing can produce rheological and material mechanical properties. Silica particles can also act as nucleating agents to influence the crystallization behavior. One studies the effect of crystalline silica on crystalline polystyrene filled with polybutylene terephthalate (polybutylene terephthalate) fibers. They found a stable fibril crystallization rate by increasing the content of polybutylene terephthalate and silica. On the other hand, no significant change in the melt crystallization temperature of the PA was found in the PA/ABS/SiO2 nanocomposites.The blending of PP with engineering plastics, such as polyesters, polyamides, and polycarbonates, may be a useful way to improve PP properties. That is, improving thermal stability, increasing stiffness, improving processability, surface finish, and dyeability. The surface-integrated nano-silica heat-generating morphologies require hybrid compatibilization for the 80/20 weight ratio of the thermal and tensile properties of the blended polyamide and polypropylene (increasedperformance). Before this work, some studies [22] that is, PA is the main component). This indicates that the interfacially constrained hydrophobic silica nanoparticles obstruct the dispersed phase; from the polymer and allowing a refinement of morphology, reducing the mixing scale can improve the tensile properties of the mixture.The main objective of the present study was to investigate the effect of nanosilica alone on the morphological, crystalline, and tensile properties of mixtures of nanosilica alone (for mixed phases with polypropylene as a matrix and ester as a filler. In particular, PA/PC or PA/nano The effect of SiO 2 and PC/nanosilica on the nucleation and crystallization effects of PP as the main component.We were able to study the determination of the nucleation kinetics of PP and the growth kinetics of the particles by means of polarization optical microscopy. DSC measures the overall crystallization kinetics.Therefore, a more detailed assessment of the nucleation and spherulite growth of PP was performed, however, the effect of nanosilica added in the second stage was not determined. The result was Akemi and Hoffman. And Huffman's crystal theory is reasonable.2 test phase2.1 Raw materialsThe polymer used in this study was a commercial product: isotactic polypropylene came from a homopolymer of polypropylene. The Frenchformula (B10FB melt flow index 2.16Kg = 15.6g / 10min at 240 °C) nylon 6 from DSM engineering plastics, Netherlands (Agulon Fahrenheit temperature 136 °C, melt flow index 240 °C 2.16kg = 5.75g / 10min ) Polycarbonate used the production waste of automotive headlamps, its melt flow index = 5g / 10min at 240 °C and 2.16kg.The silica powder TS530 is from Cabot, Belgium (about 225 m/g average particle (bone grain) about 200-300 nm in length, later called silica is a hydrophobic silica synthesis of hexamethyldisilane by gas phase synthesis. Reacts with silanols on the surface of the particles.2.2 ProcessingPP_PA and PP-PC blends and nanocomposites were hot melt mixed in a rotating twin screw extruder. Extrusion temperatures range from 180 to 240 °C. The surfaces of PP, PA, and PC were vacuumized at 80°C and the polymer powder was mixed into the silica particles. The formed particles were injected into a standard tensile specimen forming machine at 240C (3 mm thickness of D638 in the American Society for Testing Materials). Prior to injection molding, all the spherulites were in a dehumidified vacuum furnace (at a temperature of 80°C overnight). The molding temperature was 30°C. The mold was cooled by water circulation. The mixture of this combination is shown in the table.2.3 Feature Description2.31 Temperature Performance TestA PerkineElmer DSC diamond volume thermal analysis of nanocomposites. The weight of the sample is approximately 5 mg and the scanning speed is 20 °C/min during cooling and heating. The heating history was eliminated, keeping the sample at high temperature (20°C above the melting point) for three minutes. Study the sample's ultra-high purity nitrogen and calibrate the instrument with indium and tin standards.For high temperature crystallization experiments, the sample cooling rate is 60°C/min from the melt directly to the crystal reaching the temperature. The sample is still three times longer than the half-crystallization time of Tc. The procedure was deduced by Lorenzo et al. [24] afterwards.2.3.2 Structural CharacterizationScanning electron microscopy (SEM) was performed at 10 kV using a JEOL JSM 6100 device. Samples were prepared by gold plating after fracture at low temperature. Transmission electron microscopy (TEM) micrographs with a Philips cm100 device using 100 kV accelerating voltage. Ultra-low cut resection of the sample was prepared for cutting (Leica Orma).Wide-Angle X-Ray Diffraction Analysis The single-line, Fourier-type, line-type, refinement analysis data were collected using a BRUKER D8 diffractometer with copper Kα radiation (λ = 1.5405A).Scatter angles range from 10o to 25°. With a rotary step sweep 0.01° 2θ and the step time is 0.07s. Measurements are performed on the injection molded disc.This superstructure morphology and observation of spherulite growth was observed using a Leica DM2500P polarized light optical microscope (PLOM) equipped with a Linkam, TP91 thermal stage sample melted in order to eliminate thermal history after; temperature reduction of TC allowed isothermal crystallization to occur from the melt. The form is recorded with a Leica DFC280 digital camera. A sensitive red plate can also be used to enhance contrast and determine the birefringence of the symbol.2.3.3 Mechanical AnalysisTensile tests were carried out to measure the stretch rate at 10 mm/min through a Lloyd LR 10 K stretch bench press. All specimens were subjected to mechanical tests for 20 ± 2 °C and 50 ± 3% relative humidity for at least 48 hours before use. Measurements are averaged over six times.3 results3.1 Characterization by Electron MicroscopyIt is expected that PP will not be mixed with PC, PA because of their different chemical properties (polar PP and polar PC, PA) blends with 80 wt% of PP, and the droplets and matrix of PA and PC are expectedmorphologies [ 1-4] The mixture actually observed through the SEM (see Figures 1 a and b).In fact, because the two components have different polar mixtures that result in the formation of an unstable morphology, it tends to macroscopic phase separation, which allows the system to reduce its total free energy. During shearing during melting, PA or PP is slightly mixed, deformed and elongated to produce unstable slender structures that decompose into smaller spherical nodules and coalesce to form larger droplets (droplets are neat in total The size of the blend is 1 ~ 4mm.) Scanning electron microscopy pictures and PP-PC hybrid PP-PA neat and clean display left through the particle removal at cryogenic temperatures showing typical lack of interfacial adhesion of the immiscible polymer blend.The addition of 5% by weight of hydrophobic silica to the LED is a powerful blend of reduced size of the disperse phase, as can be observed in Figures 1c and D. It is worth noting that most of the dispersed phase droplets are within the submicron range of internal size. The addition of nano-SiO 2 to PA or PC produces finer dispersion in the PP matrix.From the positional morphology results, we can see this dramatic change and the preferential accumulation at the interface of silica nanoparticles, which can be clearly seen in FIG. 2 . PP, PA part of the silicon is also dispersed in the PP matrix. It can be speculated that thisformation of interphase nanoparticles accumulates around the barrier of the secondary phase of the LED, thus mainly forming smaller particles [13, 14, 19, 22]. According to fenouillot et al. [19] Nanoparticles are mixed in a polymer like an emulsifier; in the end they will stably mix. In addition, the preferential location in the interval is due to two dynamic and thermodynamic factors. Nanoparticles are transferred to the preferential phase, and then they will accumulate in the interphase and the final migration process will be completed. Another option is that there isn't a single phase of optimization and the nanoparticles will be set permanently in phase. In the current situation, according to Figure 2, the page is a preferential phase and is expected to have polar properties in it.3.2 Wide-angle x-ray diffractionThe polymer and silica incorporate a small amount of nanoparticles to modify some of the macroscopic properties of the material and the triggered crystal structure of PP. The WAXD experiment was performed to evaluate the effect of the incorporation of silica on the crystalline structure of the mixed PP.Isotactic polypropylene (PP) has three crystalline forms: monoclinic, hexagonal, and orthorhombic [25], and the nature of the mechanical polymer depends on the presence of these crystalline forms. The metastable B form is attractive because of its unusual performance characteristics, including improved impact strength and elongation atbreak.The figure shows a common form of injection molding of the original PP crystal, reflecting the appearance at 2θ = 14.0, 16.6, 18.3, 21.0 and 21.7 corresponding to (110), (040), (130), (111) and (131) The face is an α-ipp.20% of the PA incorporation into PP affects the recrystallization of the crystal structure appearing at 2θ = 15.9 °. The corresponding (300) surface of the β-iPP crystal appears a certain number of β-phases that can be triggered by the nucleation activity of the PA phase in PP (see evidence The following nucleation) is the first in the crystalline blend of PA6 due to its higher crystallization temperature. In fact, Garbarczyk et al. [26] The proposed surface solidification caused by local shear melts the surface of PA6 and PP and forms during the injection process, promoting the formation of β_iPP. According to quantitative parameters, KX (Equation (1)), which is commonly used to evaluate the amount of B-crystallites in PP including one and B, the crystal structure of β-PP has 20% PP_PA (110), H(040) and Blends of H (130) heights (110), (040) and (130). The height at H (300) (300) for type A peaks.However, the B characteristic of 5 wt% silica nanoparticles incorporated into the same hybrid LED eliminates reflection and reflection a-ipp retention characteristics. As will be seen below, the combination of PA and nanosilica induces the most effective nucleatingeffect of PP, and according to towaxd, this crystal formation corresponds to one PP structure completely.The strong reductive fracture strain observations when incorporated into polypropylene and silica nanoparticles (see below) cannot be correlated to the PP crystal structure. In fact, the two original PP and PP_PA_SiO2 hybrids contain α_PP but the original PP has a very high form of failure when the strain value.On the other hand, PP-PC and PP-PC-Sio 2 blends, through their WAXD model, can be proven to contain only one -PP form, which is a ductile material.3.3 Polarized Optical Microscopy (PLOM)To further investigate the effect of the addition of two PAs, the crystallization behavior of PC and silica nanoparticles on PP, the X-ray diffraction analysis of its crystalline structure of PP supplements the study of quantitative blends by using isothermal kinetic conditions under a polarizing microscope. The effect of the composition on the nucleation activity of PP spherulite growth._Polypropylene nucleation activityThe nucleation activity of a polymer sample depends on the heterogeneity in the number and nature of the samples. The second stage is usually a factor in the increase in nucleation density.Figure 4 shows two isothermal crystallization temperatures for thePP nucleation kinetics data. This assumes that each PP spherulite nucleates in a central heterogeneity. Therefore, the number of nascent spherulites is equal to the number of active isomerous nuclear pages, only the nucleus, PP-generated spherulites can be counted, and PP spherulites are easily detected. To, while the PA or PC phases are easily identifiable because they are secondary phases that are dispersed into droplets.At higher temperatures (Fig. 4a), only the PP blend inside is crystallized, although the crystals are still neat PP amorphous at the observed time. This fact indicates that the second stage of the increase has been able to produce PP 144 °C. It is impossible to repeat the porous experiment in the time of some non-homogeneous nucleation events and neat PP exploration.The mixed PP-PC and PP-PC-SiO 2 exhibited relatively low core densities at 144 °C, (3 105 and 3 106 nuc/cm 3) suggesting that either PC nanosilica can also be considered as good shape Nuclear agent is used here for PP.On the other hand, PA, himself, has produced a sporadic increase in the number of nucleating events in PP compared to pure PP, especially in the longer crystallization time (>1000 seconds). In the case of the PP-PA _Sio 2 blend, the heterogeneous nucleation of PP is by far the largest of all sample inspections. All the two stages of the nucleating agent combined with PA and silica are best employed in this work.In order to observe the nucleation of pure PP, a lower crystallization temperature was used. In this case, observations at higher temperatures found a trend that was roughly similar. The neat PP and PP-PC blends have small nucleation densities in the PP-PC-SiO 2 nanocomposite and the increase also adds further PP-PA blends. The very large number of PP isoforms was rapidly activated at 135°C in the PP-PA nanoparticle nanometer SiO 2 composites to make any quantification of their numbers impossible, so this mixed data does not exist from Figure 4b.The nucleation activity of the PC phase of PP is small. The nucleation of any PC in PP can be attributed to impurities that affect the more complex nature of the PA from the PC phase. It is able to crystallize at higher temperatures than PP, fractional crystallization may occur and the T temperature is shifted to much lower values (see References [29-39]. However, as DSC experiments show that in the current case The phase of the PA is capable of crystallizing (fashion before fractionation) the PP matrix, and the nucleation of PP may have epitaxy origin.The material shown in the figure represents a PLOAM micrograph. Pure PP has typical α-phase negative spherulites (Fig. 5A) in the case of PP-PA blends (Fig. 5B), and the PA phase is dispersed with droplets of size greater than one micron (see SEM micrograph, Fig. 1) . We could not observe the spherulites of the B-phase type in PP-PA blends. Even according to WAXD, 20% of them can be formed in injection moldedspecimens. It must be borne in mind that the samples taken using the PLOAM test were cut off from the injection molded specimens but their thermal history (direction) was removed by melting prior to melting for isothermal crystallization nucleation experiments.The PA droplets are markedly enhanced by the nucleation of polypropylene and the number of spherulites is greatly increased (see Figures 4 and 5). Simultaneously with the PP-PA blend of silica nanoparticles, the sharp increase in nucleation density and Fig. 5C indicate that the size of the spherulites is very small and difficult to identify.The PP-PC blends showed signs of sample formation during the PC phase, which was judged by large, irregularly shaped graphs. Significant effects: (a) No coalesced PC phase, now occurring finely dispersed small droplets and (B) increased nucleation density. As shown in the figure above, nano-SiO 2 tends to accumulate at the interface between the two components and prevent coalescence while promoting small disperse phase sizes.From the nucleation point of view, it is interesting to note that it is combined with nanosilica and as a better nucleating agent for PP. Combining PCs with nanosilica does not produce the same increase in nucleation density.Independent experiments (not shown here) PP _ SiO 2 samplesindicate that the number of active cores at 135 °C is almost the same as that of PP-PC-SiO2 intermixing. Therefore, silica cannot be regarded as a PP nucleating agent. Therefore, the most likely explanation for the results obtained is that PA is the most important reason for all the materials used between polypropylene nucleating agents. The increase in nucleation activity to a large extent may be due to the fact that these nanoparticles reduce the size of the PA droplets and improve its dispersion in the PP matrix, improving the PP and PA in the interfacial blend system. Between the regions. DSC results show that nano-SiO 2 is added here without a nuclear PA phase.4 Conclusion5% weight of polypropylene/hydrophobic nanosilica blended polyamide and polypropylene/polycarbonate (80E20 wt/wt) blends form a powerful LED to reduce the size of dispersed droplets. This small fraction of reduced droplet size is due to the preferential migration of silica nanoparticles between the phases PP and PA and PC, resulting in an anti-aggregation and blocking the formation of droplets of the dispersed phase.The use of optical microscopy shows that the addition of PA, the influence of PC's PA-Sio 2 or PC-Sio 2 combination on nucleation, the nucleation density of PP polypropylene under isothermal conditions is in the following approximate order: PP <PP-PC <PP -PC-SiO 2<<PP-PA<<< PP-PA-SiO 2. PA Drip Nucleation PP Production of nucleation densities at isothermal temperatures is higher than with PC or PC Sio 2D. When nanosilica is also added to the PP-PA blend, the dispersion-enhanced mixing of the enhanced nanocomposites yields an intrinsic factor PP-PA-Sio2 blend that represents a PA that is identified as having a high nucleation rate, due to nanoseconds Silicon oxide did not produce any significant nucleation PP. PLOAM was found to be a more sensitive tool than traditional cooling DSC scans to determine differences in nucleation behavior. The isothermal DSC crystallization kinetics measurements also revealed how the differences in nucleation kinetics were compared to the growth kinetic measurements.Blends (and nanocomposites of immiscible blends) and matrix PP spherulite assemblies can grow and their growth kinetics are independent. The presence of a secondary phase of density causes differences in the (PA or PC) and nanosilica nuclei. On the other hand, the overall isothermal crystallization kinetics, including nucleation and growth, strongly influence the nucleation kinetics by PLOAM. Both the spherulite growth kinetics and the overall crystallization kinetics were successfully modeled by Laurie and Huffman theory.Although various similarities in the morphological structure of these two filled and unfilled blends were observed, their mechanical properties are different, and the reason for this effect is currently being investigated.The addition of 5% by weight of hydrophobic nano-SiO 2 resulted in breaking the strain-enhancement of the PP-PC blend and further weakening the PP-PA blend.中文译文纳米二氧化硅对PP-PC和PP-PA共混物的成核,结晶和热塑性能的影响Laoutid F, Estrada E, Michell R M, et al摘要80(wt%)聚丙烯与20(wt %)聚酰胺和聚碳酸酯有或没有添加5%纳米二氧化硅通过熔融混合制备不混溶的共聚物。
硅基仿生 工艺流程
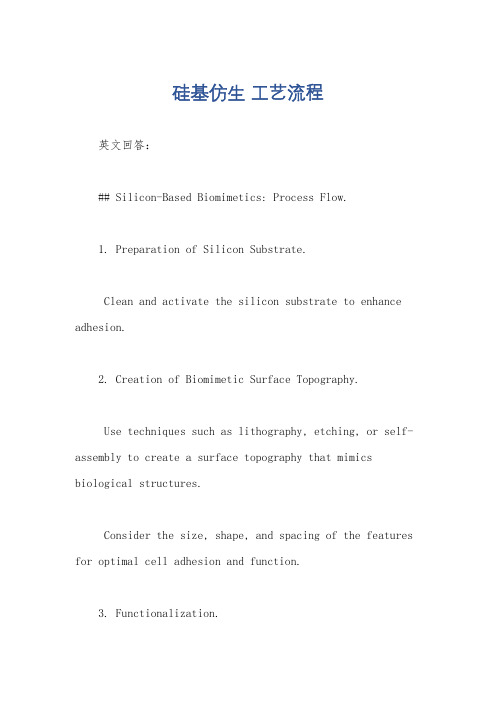
硅基仿生工艺流程英文回答:## Silicon-Based Biomimetics: Process Flow.1. Preparation of Silicon Substrate.Clean and activate the silicon substrate to enhance adhesion.2. Creation of Biomimetic Surface Topography.Use techniques such as lithography, etching, or self-assembly to create a surface topography that mimics biological structures.Consider the size, shape, and spacing of the features for optimal cell adhesion and function.3. Functionalization.Introduce functional groups to the surface to promote specific cell interactions.This can involve coating with extracellular matrix proteins, growth factors, or specific ligands.4. Cell Seeding.Dispense the desired cell type onto the biomimetic substrate.Optimize cell density and seeding parameters to achieve desired cell attachment and proliferation.5. Culture.Culture the cells on the biomimetic substrate under appropriate conditions.Provide nutrients, growth factors, and other essential factors for cell viability and function.6. Assessment.Evaluate cell adhesion, proliferation, differentiation, and overall functionality.Use techniques such as microscopy, assays, and electrophysiology to study cell response.7. Optimization.Based on the assessment results, refine the biomimetic surface topography, functionalization, or culture parameters.Iterate the process to achieve optimal cell behavior.中文回答:硅基仿生工艺流程。
常压干燥制备疏水性 SiO2气凝胶
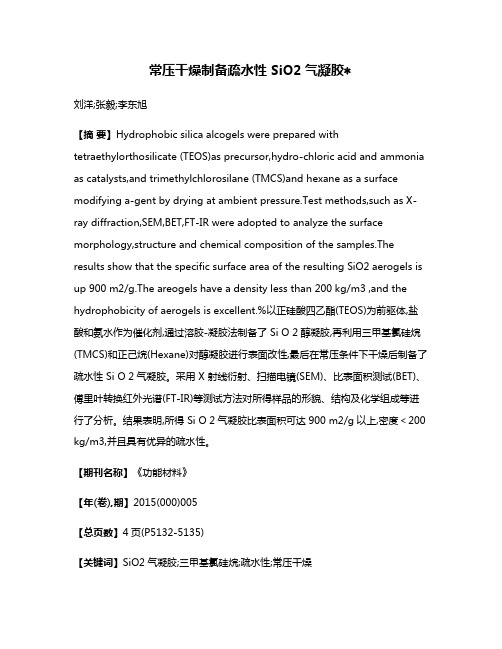
常压干燥制备疏水性 SiO2气凝胶∗刘洋;张毅;李东旭【摘要】Hydrophobic silica alcogels were prepared with tetraethylorthosilicate (TEOS)as precursor,hydro-chloric acid and ammonia as catalysts,and trimethylchlorosilane (TMCS)and hexane as a surface modifying a-gent by drying at ambient pressure.Test methods,such as X-ray diffraction,SEM,BET,FT-IR were adopted to analyze the surface morphology,structure and chemical composition of the samples.The results show that the specific surface area of the resulting SiO2 aerogels is up 900 m2/g.The areogels have a density less than 200 kg/m3 ,and the hydrophobicity of aerogels is excellent.%以正硅酸四乙酯(TEOS)为前驱体,盐酸和氨水作为催化剂,通过溶胶-凝胶法制备了 Si O 2醇凝胶,再利用三甲基氯硅烷(TMCS)和正己烷(Hexane)对醇凝胶进行表面改性,最后在常压条件下干燥后制备了疏水性 Si O 2气凝胶。
采用 X 射线衍射、扫描电镜(SEM)、比表面积测试(BET)、傅里叶转换红外光谱(FT-IR)等测试方法对所得样品的形貌、结构及化学组成等进行了分析。
结果表明,所得 Si O 2气凝胶比表面积可达900 m2/g以上,密度<200 kg/m3,并且具有优异的疏水性。
卡博特气相二氧化硅生产流程
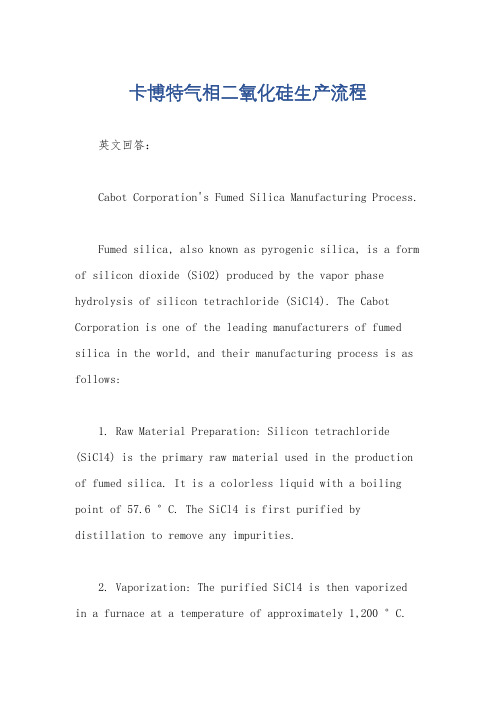
卡博特气相二氧化硅生产流程英文回答:Cabot Corporation's Fumed Silica Manufacturing Process.Fumed silica, also known as pyrogenic silica, is a form of silicon dioxide (SiO2) produced by the vapor phase hydrolysis of silicon tetrachloride (SiCl4). The Cabot Corporation is one of the leading manufacturers of fumed silica in the world, and their manufacturing process is as follows:1. Raw Material Preparation: Silicon tetrachloride (SiCl4) is the primary raw material used in the production of fumed silica. It is a colorless liquid with a boiling point of 57.6 °C. The SiCl4 is first purified bydistillation to remove any impurities.2. Vaporization: The purified SiCl4 is then vaporizedin a furnace at a temperature of approximately 1,200 °C.This vaporization process converts the liquid SiCl4 into a gas.3. Hydrolysis: The SiCl4 gas is then introduced into a reaction chamber where it reacts with water vapor to form silicon dioxide (SiO2) and hydrogen chloride (HCl). The reaction is as follows:SiCl4 + 2H2O → SiO2 + 4HCl.4. Agglomeration: The SiO2 particles produced in the hydrolysis reaction are very small, with an average diameter of approximately 10 nanometers. These small particles can agglomerate to form larger particles, which are then collected on a filter.5. Drying and Annealing: The collected SiO2 particles are then dried and annealed to remove any residual moisture and to improve their physical properties.The final product is a white, fluffy powder with a high surface area and a low bulk density. Fumed silica is usedin a variety of applications, including as a thickening agent, a reinforcing agent, and a polishing agent.中文回答:卡博特气相二氧化硅生产流程。
溶胶凝胶法制备氧化硅气凝胶的工艺流程
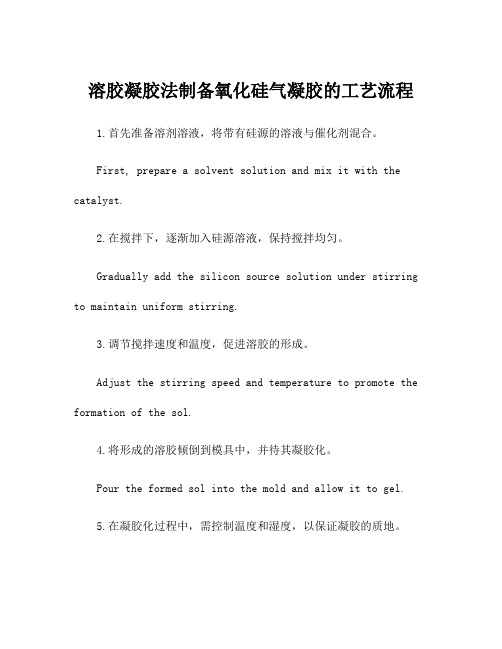
溶胶凝胶法制备氧化硅气凝胶的工艺流程1.首先准备溶剂溶液,将带有硅源的溶液与催化剂混合。
First, prepare a solvent solution and mix it with the catalyst.2.在搅拌下,逐渐加入硅源溶液,保持搅拌均匀。
Gradually add the silicon source solution under stirring to maintain uniform stirring.3.调节搅拌速度和温度,促进溶胶的形成。
Adjust the stirring speed and temperature to promote the formation of the sol.4.将形成的溶胶倾倒到模具中,并待其凝胶化。
Pour the formed sol into the mold and allow it to gel.5.在凝胶化过程中,需控制温度和湿度,以保证凝胶的质地。
During the gelation process, it is necessary to controlthe temperature and humidity to ensure the quality of the gel.6.将凝胶放入干燥箱中,进行干燥处理。
Put the gel into the drying oven for drying.7.根据需要,可以对氧化硅气凝胶进行热处理,以进一步改变其性质。
If necessary, the silica aerogel can be heat-treated to further change its properties.8.最后对氧化硅气凝胶进行表面处理,以提高其稳定性和耐久性。
Finally, the silica aerogel is subjected to surface treatment to improve its stability and durability.9.溶胶凝胶法制备氧化硅气凝胶的工艺流程包括多个步骤。
石榴石型固态电解质
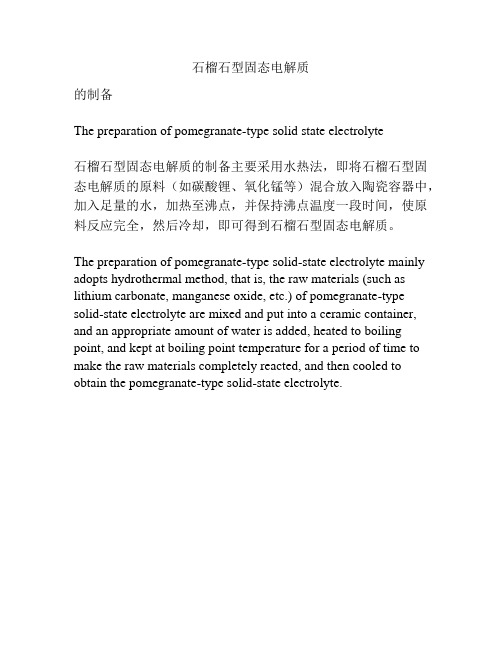
石榴石型固态电解质
的制备
The preparation of pomegranate-type solid state electrolyte
石榴石型固态电解质的制备主要采用水热法,即将石榴石型固态电解质的原料(如碳酸锂、氧化锰等)混合放入陶瓷容器中,加入足量的水,加热至沸点,并保持沸点温度一段时间,使原料反应完全,然后冷却,即可得到石榴石型固态电解质。
The preparation of pomegranate-type solid-state electrolyte mainly adopts hydrothermal method, that is, the raw materials (such as lithium carbonate, manganese oxide, etc.) of pomegranate-type solid-state electrolyte are mixed and put into a ceramic container, and an appropriate amount of water is added, heated to boiling point, and kept at boiling point temperature for a period of time to make the raw materials completely reacted, and then cooled to obtain the pomegranate-type solid-state electrolyte.。
氨基功能化介孔氧化硅材料的制备 2

氨基功能化介孔氧化硅材料的制备摘要介孔氧化硅材料由于其较大的孔容和比表面积,较好的生物相容性和无毒性等优点,受到越来越多研究者的关注。
有机-无机介孔材料也称为PMOs(Periodic Mesoporous Organosilicas)是采用共缩聚的方法以桥联的有机硅酯作为硅源前体,将有机基团键合在材料的骨架中,可以使有机基团更均匀地分布在材料的骨架中并且不会堵塞孔道。
PMOs 材料规则的孔道分布、可调的孔道微环境、丰富的有机基团等性质赋予了其潜在的应用前景,尤其在药物负载中显示了独特性能。
双模型介孔材料(BMMs)是一种新型介孔材料,它具有双孔道结构:3 nm 左右的蠕虫状一级孔与10-30 nm左右的球形颗粒堆积孔。
由于BMMs有别于单一孔道介孔材料,具有结构可控和粒度可控等许多独特性质,通过进一步表面改性,能够针对特定的药物分子,尤其是不溶性药物分子进行装载与可控释放,具有很好的专一性。
关键词:双模型介孔材料;氨基功能化;载药AbstractMesoporous silica materials due to its larger surface area, pore volume, advantages of good biocompatibility and non-toxic got more and more attention from researchers. Organic-inorganic mesoporous materials is also known as PMOs (Periodic Mesoporous Organosilicas) is using the copolycondensation method to bridging the silicone ester as a silicon source precursor, The organic group bonded in the skeleton material can make the organic groups more evenly distributed in the frame of material and will not block channel. PMOs material rules of channel distribution, adjustable pore micro environment, abundant organic groups leading to its potential application, especially shows the unique performancei n drug load. Bimodal mesoporous material (BMMs) is a new mesoporous material consisting of worm-like mesopores of 3nm as well as large inter-particles pores around 10-30 nm. Different from mesoporous materials with only one pore distribution, BMMs could realize the loading and controlled release of specific drug molecules, especially for the insoluble drugs, through surface modification, due to the unique characteristics such as the controllable structure and particles size.Keywords: Bimodal mesoporous material; Amino functionalization; drug1. 绪论1.1介孔分子筛的概述在世界经济和科学技术高速发展的今天,材料与能源、信息并列为现代科学技术的三大支柱,其作用和意义尤为重要。
利用简单模板制备多孔二氧化硅

2009年10月 The Chinese Journal of Process Engineering Oct. 2009收稿日期:2009−04−07,修回日期:2009−06−02基金项目:国家高技术研究发展计划(863)基金资助项目(编号:2007AA03Z439)作者简介:刘海弟(1976−),男,陕西省杨凌市人,博士,助理研究员,从事功能材料研究;陈运法,通讯联系人,E-mail: yfchen@.利用简单模板制备多孔二氧化硅刘海弟, 岳仁亮, 张冬海, 吴镇江, 陈运法(中国科学院过程工程研究所多相复杂系统国家重点实验室,北京 100190)摘 要:以简单有机模板为致孔剂、正硅酸乙酯(TEOS)为硅源制备了多孔SiO 2,考察了模板剂加入量对样品比表面和孔容的影响,测定了样品的比表面和孔结构,提出了简单有机模板对多孔SiO 2的致孔机理,并与以碱性硅溶胶为硅源对比,验证了该机理. 结果表明,简单有机模板添加量为模板剂/TOES =0.3 mg/mL 时,样品比表面积可提高至650 m 2/g 以上,孔容大于1.0 mL/g ,孔道为无规则结构. 该多孔结构的产生很可能是因为简单有机模板和TEOS 的聚合物发生分子级混合,从而在凝胶和煅烧过程中使样品中产生了丰富的孔道. 关键词:多孔二氧化硅;溶胶−凝胶;模板中图分类号:TQ050.4+21 文献标识码:A 文章编号:1009−606X(2009)05−1005−061 前 言多孔材料由于其巨大的比表面、丰富的孔道、优异的吸附性能而经常用作催化剂载体、污染物吸附剂、环境净化功能材料、高效液相色谱填料、气体分离材料、绝热材料等. 其中多孔SiO 2由于化学性质相对稳定、不燃烧、耐酸等特点而长期为材料制备领域所重视. 1992年Kresge 等[1]和Beck 等[2]采用十六烷基三甲基溴化铵(Cetyl-trimethyl Ammonium Bromide, CTAB)为模板剂成功制备出了孔径2 nm 左右的高比表面介孔分子筛,命名为M41S 系列,引起了各国研究人员的广泛关注. 此后合成了一系列介孔SiO 2材料,Huo 等[3,4]在酸性条件下利用Gemini(Cn-s-m)型表面活性剂合成了笼状三维六方结构的SBA-n 系列分子筛;Tanev 等[5−7]利用长链伯胺和长链二胺为模板剂合成孔壁较厚的介孔分子筛;Zhao 等[8,9]以较高分子量的嵌段共聚物[Poly(ethylene oxide)−Poly(propylene oxide)−Poly(ethylene oxide), PEO −PPO −PEO]为模板合成了孔径大且孔壁厚的介孔分子筛SBA-15,孔径可达30 nm 可调,壁厚达6 nm ,水热稳定性较高. 介孔SiO 2以其在新型控释药物、高选择性催化剂等方面广阔的应用前景而仍是材料制备科学研究中的热点. 纵观这些研究结果可知,介孔材料的突出特点在于其规则的孔道结构,而孔结构依赖于模板剂,模板剂包括阳离子表面活性剂、长链有机胺、嵌段共聚物等,价格昂贵,对大量制备介孔材料带来了一定困难. 同时,在反应物分子尺寸小且反应选择性要求不高的催化反应中(如水中苯酚的催化降解、NO x 催化氧化吸收等),高度规则的孔道和均一的孔径是不必要的,所以采用廉价易得的模板剂制备高比表面的无规则多孔SiO 2具有一定的意义.采用硅酸四乙酯(Tetra-ethoxy-silane, TEOS)为硅源制备SiO 2薄膜和块体材料的研究已有很多报道[10−20]. 为避免SiO 2凝胶在干燥过程中因孔道塌陷而导致比表面损失,很多研究者[15,16,19]采用超临界干燥方法得到多孔产品,否则所得样品比表面不高[18],这对设备提出了很高的要求,且存在难以工业放大的问题. 王娟等[16]采用聚乙二醇[Poly(ethylene glycol), PEG]为添加剂获得了多孔SiO 2薄膜,但仍采用超临界法对其进行干燥. 孙继红等[20]对SiO 2−PEG 凝胶体系进行了研究,认为PEG 能限制TEOS 的水解反应且有利于保持SiO 2空间网络结构,但由于采用单一的碱催化水解,使凝胶过程耗时较长且需要抽真空干燥以去除模板.本研究采用先酸催化TEOS 聚合、再碱催化凝胶的方法,缩短了SiO 2凝胶的形成时间,同时考察并比较了4种简单有机模板(PEG2000和PEG4000、聚乙烯吡咯烷酮、松香)对多孔SiO 2材料形成的影响. 在避免使用超临界干燥和抽真空干燥的前提下,制备了比表面积大于650 m 2/g 、且孔道发达的SiO 2样品,为环境净化研究领域提供了一种相对廉价的高比表面积多孔SiO 2载体制备方法. 对材料的生成机理进行了探讨,并利用硅溶胶为硅源进行了对比实验,证明硅源是否以分子或多分子状态为凝胶起点对能否得到高比表面积的多孔SiO 2样品至关重要.2 实 验2.1 主要原料和试剂无水乙醇EtOH(CH 3CH 2OH)、正硅酸乙酯TEOS [Si(OCH 2CH 3)4]、盐酸[HCl 37%(ω)]、氨水[NH 3⋅H 2O28%(ω)]、氯化铵(NH4Cl)、聚乙二醇(PEG, 分子量2000和4000)、聚乙烯吡咯烷酮(PVP, K30)均为分析纯,北京益利化学试剂公司. 松香购自北京化学试剂公司,乙醇不溶物<0.03%(ω), 灰分<0.03%(ω). 碱性硅溶胶购自北京飞龙马防水材料公司,pH 9, SiO2 30%(ω),粒径10∼20 nm,密度1.2 g/cm3, Na2O<0.3%(ω). 实验用水均为去离子水.2.2 实验方法2.2.1 TEOS预聚合取10 mL TEOS溶于20 mL无水乙醇中,加入0.3 mL浓盐酸和0.6 mL去离子水,冷凝回流和磁力搅拌下在85℃水浴中反应3 h,将所得透明均一溶液取出待用,命名为PTEOS.2.2.2 凝胶和多孔SiO2制备将称量好的简单有机模板溶于PTEOS溶液中,剧烈搅拌下20 s内加入4 mL浓氨水,将所得透明均一溶液静置,1∼10 min内凝胶,凝胶强度较好,具有一定弹性. 将凝胶在60℃烘箱中烘干48 h,再于马弗炉中煅烧(室温∼650℃升温速率2℃/min, 650℃保持6 h),得多孔SiO2材料,命名为Name-x,Name为模板剂,如PEG2000, PEG4000, PVP和Rosin(松香),x为模板剂/TEOS值(g/mL).2.2.3 以硅溶胶为硅源制备多孔SiO2将称量好的PEG2000溶于10 mL硅溶胶与10 mL 水的混合物中,剧烈搅拌下10 s内加入4 mL 23%(ω) NH4Cl溶液,将所得均一溶胶静置,4 h内成为凝胶. 将凝胶在60℃烘箱中烘干48 h,再于马弗炉中煅烧(室温∼650℃升温速率2℃/min, 650℃保持6 h),得多孔SiO2材料,命名为PEG2000-sol-y, y为模板剂/硅溶胶比值(g/mL).2.3 样品分析和表征将多孔SiO2样品在300℃下抽真空处理3 h,用Beckman Coulter SA3100型氮吸附比表面仪测定其比表面积和孔容,用JSM-6700F型场发射扫描电子显微镜分析外形和断面形貌,用Tecnai G2 20 ST型透射电子显微镜观察颗粒形貌和聚结状况.3 结果和讨论3.1 TEOS的预水解在酸催化条件下,TEOS可在醇水环境中进行水解和缩聚反应转变为二聚物、多聚物、齐聚物及其他更复杂的聚合物,决定反应产物形态的重要因素是反应体系中的水量. 根据化学计量式,1分子TEOS水解需要4分子水,但当2个Si⎯OH基团间脱水缩和时又会放出1分子水,所以如果要生成主链为⎯O⎯Si⎯O而支链全为Si⎯OC2H5的聚合物,需要2倍于TEOS物质的量的水. 根据Kamiya等[21]提出的 TEOS−H2O−C2H5OH体系三元相图,选择反应物配比在形成透明均一溶液和溶胶的范围内,此时TEOS:H2O:EtOH摩尔比为1:1:8. 实验发现,当TEOS:EtOH:HCl的摩尔比为1:8:0.06时,反应体系中H2O:TEOS摩尔比可在1:1∼2.5:1间选择. 但如果第一步中加水量过大,第二步的碱催化凝胶过程中加入的氨水会使体系产生团絮状凝胶,而不是透明均一的整体凝胶,所以反应中选择H2O:TEOS摩尔比为1:1.3.2 采用不同简单有机模板时样品的比表面积实验中考察了PEG2000, PEG4000, PVP和松香几种模板剂对样品比表面积的影响,结果如表1所示.表 1 不同用量不同模板剂所得多孔SiO2的比表面积Table 1 BET specific surface area of porous silica obtainedunder different templates and various amounts (m2/g) Template/TEOS(g/mL)PEG2000PEG4000 PVP(K30)Rosin0 221−−−0.1 608 553 526 5710.3 765 685 667 6910.5 776 − 694 7671 636 −−−Note: Not conducted.从表1可见,加入模板剂明显提高了SiO2样品的比表面积,提高程度与模板剂种类关系不大,只与其用量有关. 不添加任何模板剂时,所得SiO2样品比表面积为221 m2/g,这是一般溶胶−凝胶法所得SiO2常见的比表面积. 加入0.1 g/mL模板剂时,样品比表面积显著提高至520 m2/g以上,进一步提高用量至0.3和0.5 g/mL 时,样品比表面积分别增大至660和700 m2/g左右. 当模板剂用量提高至1 g/mL时,样品比表面积反而有所减小. 可见,简单有机模板提高SiO2比表面积的能力有限,用量太高比表面积反而下降. 同时可知,简单有机模板不能像CTAB那样将SiO2的比表面积提高至1000m2/g以上,原因可能是模板剂在SiO2凝胶中无规分散,没有像CTAB胶束那样使SiO2产生微细规则的孔道.由于PEG4000分子量较大,其用量增大时体系粘度过大不易搅拌,所以未尝试0.5 g/mL和更大用量. 为使样品有较大比表面积而模板剂用量不致过大,选择0.3∼0.5 g/mL用量较合适. 比较4种模板剂可知,松香对乙醇溶液中的水极其敏感,水量稍多则立即产生絮凝沉淀;而PVP虽然溶解性很好,但价格昂贵;PEG4000溶解较难且溶液粘度太高,因此PEG2000是最好的模板剂.3.3 模板剂添加量对样品孔结构的影响以PEG2000为模板剂,研究了不同用量下产品的第5期 刘海弟等:利用简单模板制备多孔二氧化硅 1007孔结构. 表2给出了各样品的比表面积、总孔容、介孔孔容和平均孔径,可知未加入模板剂时样品比表面积低、平均孔径较大、孔容较小,孔道可能是SiO 2的粒间孔及SiO 2凝胶干燥过程产生的孔. 随模板剂加入量增加,样品比表面积不断提高、平均孔径减小、孔容明显增大. 可以推断,模板剂使样品的孔道数大大增加,内部孔道更加发达,且介孔的孔容也有所增多,这可能是包嵌在SiO 2凝胶中的模板剂起到了“撑出”孔道的作用.表2 不同模板剂用量所得多孔SiO 2样品的比表面和累积孔容Table 2 BET specific surface area and pore structure results of porous silica samples under various template amountsSampleTotal specific BET surface area,S BET (m 2/g)Cumulative adsorption mesopore volume, V meso 1) (m 2/g) Cumulative adsorption volume, V BJH 2)(mL/g) Average pore size (nm) PEG2000-0 221 0.36 0.55 10.1 PEG2000-0.1 608 0.38 0.76 5.00 PEG2000-0.3 765 0.48 1.08 5.67 PEG2000-0.5 776 0.51 1.16 5.98 Note: 1) Pore size 1.5∼50 nm; 2) Pore size 1.5∼200 nm. 图1给出了各样品在77 K 下对N 2的吸附/脱附曲线. 根据IUPAC 划分方法,各样品的等温吸附线均可大致划为IV 型,说明样品中含有导致N 2吸附过程中发生毛细管凝结的介孔. 同样可知,随模板剂用量提高,样品的N 2吸附容量也逐渐提高,说明添加模板剂使SiO 2中的孔道更加丰富、孔容提高.图1 不同模板剂用量下SiO 2样品的N 2吸附−脱附等温线Fig.1 N 2 adsorption −desertion isotherms of silica samples图2为各样品的孔分布曲线,PEG2000-0中既有微孔也有介孔,但孔容较低,可能是因为这些孔道来自SiO 2一次粒子的无规则堆积,因此孔径分布很宽. 而PEG2000-0.1, PEG2000-0.3和PEG2000-0.5的孔分布曲线均在9 nm 附近出现了一个宽峰,介孔范围的孔容显著提高,可见添加模板剂对提高介孔孔容很有效;同时3样品小于2 nm 的孔容比PEG2000-0增加,可知模板剂也导致产生了更多微孔. 从以上结果推断,添加简单有机模板剂对微孔和介孔孔容的提高都有明显作用. 同时可知,虽然添加的模板剂明显使孔道增多、孔容增大,但孔分布依然较宽,孔结构仍然无序. 这再次说明,简单有机模板不能得到类似分子筛那样有序的孔道结构和狭窄的孔径分布.图2 不同模板剂用量下SiO 2样品的孔分布曲线 Fig.2 Pore size distributions of silica samples3.4 样品的SEM 分析图3给出了样品PEG2000-0∼0.5颗粒断面的SEM 照片. 可以看出,没有加入模板剂的样品具有平滑的断面,其上只有少量裂缝和孔隙,而加入模板剂的各样品的断面为丰富的多孔结构,这与比表面积及孔分布分析完全一致. 对比表面积有贡献的孔道一般不易在SEM 分析中看到,综合SEM 分析和BET 分析结果可以看出,模板剂导致样品中产生了大量孔隙,不但增加了对比表面积有重大贡献的孔道的数量,甚至产生了SEM 可以分辨的孔隙,所以样品的孔容也明显提高. 3.5 样品的TEM 分析图4给出了样品PEG2000-0和PEG2000-0.5的TEM 照片. 由图4(a)可以看出,没有加入模板剂的样品PEG2000-0是由20 nm 左右的类球形SiO 2颗粒堆积而成的,可以推断其孔道主要是无规则堆积的SiO 2颗粒的粒间孔及SiO 2凝胶干燥过程中产生的孔,因此PEG2000-0的比表面积小、孔容低、平均孔径较大. 从图4(b)可知,加入模板剂的样品PEG2000-0.5颗粒形貌和聚结方式都有所改变,不再有20 nm 左右的球形SiO 2粒子,而是由粒径更加细小的无定型絮状颗粒无规则堆积而成,可见添加PEG 明显改变了SiO 2颗粒的形貌,使其从类0.00.20.40.60.8 1.00100200300400500600700800A d s o r b e d v o l u m e (m L /g )Relative pressure, p/p 02468101214160.00.20.40.60.81.01.21.4P o r e v o l u m e , D v (m L /g )Pore size (nm)1008 过 程 工 程 学 报 第9卷(a) PEG2000-0 (b) PEG2000-0.1 (c) PEG2000-0.3 (d) PEG2000-0.5图3 样品的SEM 照片Fig.3 SEM images of samples(a) PEG2000-0 (b) PEG2000-0.5图4 样品的TEM 照片Fig.4 TEM images of samples球形变成了无定型絮状结构. 原因可能是PEG 分子和PTEOS 分子链互相缠绕,导致SiO 2粒子在形成过程中与PEG 分子链互相穿插,进而在灼烧后形成了由微小的无定型絮状SiO 2组成的疏松多孔的微观结构,因此其比表面积和孔容都明显增大. 3.6 模板剂对二氧化硅的致孔机理简单有机模板剂对SiO 2的致孔机理可表述为:当简单有机模板溶解于PTEOS 的乙醇溶液时,会形成澄清透明的均一溶液,其中模板剂分子和PTEOS 分子链之间达到分子级别的均匀混合. 当把氨水加入其中并立即混合均匀时,氨水会导致体系中PTEOS 分子链上剩余乙氧基水解和缩合,从而使PTEOS 分子链间的搭接点发生连接,从而产生大型空间网络状结构,使体系在很短时间内失去流动性而形成具有较高强度的凝胶;在图5 PEG 模板的致孔机理Fig.5 Pore formation mechanism of PEG第5期 刘海弟等:利用简单模板制备多孔二氧化硅1009凝胶形成的同时,高度分散的有机物模板被“冻结”在凝胶块中,使凝胶成为有机−无机杂化材料,实现了有机物在凝胶中分子级别的均匀分布. 将该凝胶在高温下灼烧除去高分子模板,便得到了具有发达无规则孔道结构的SiO2材料. 机理如图5所示(以PEG为例).松香的情况略有不同. 松香是分子量300左右的天然树脂酸,而不是PEG和PVP那样的链状高分子. 松香在乙醇中有较好的溶解性,当PTEOS在氨的作用下发生凝胶时,松香分子同样高度分散在凝胶中,所以也可以在SiO2凝胶中起到“撑起”孔道的作用. 根据该机理,只要能溶于乙醇、能与PTEOS混溶,且在SiO2凝胶烘干过程中不会液化或挥发的有机物均可在本实验中作为模板使用.碱性硅溶胶也具有很好的凝胶特性,实验以碱性硅溶胶作为硅源进行对比. 与PTEOS溶液不同的是,碱性硅溶胶中的SiO2胶粒粒径为10∼20 nm,而胶粒间可能存在具有一定聚合交联能力的聚硅酸链,因此当酸性物质(如NH4Cl)与硅溶胶发生作用时,硅溶胶也可发生类似PTEOS的凝胶反应,但凝胶过程是以10∼20 nm的SiO2颗粒为基本单位的,而PTEOS则以聚合的硅氧烷分子链为凝胶的基本单位.表3给出了以碱性硅溶胶为硅源时的实验结果,可知添加模板剂PEG2000的样品比表面积没有明显提高,都在250 m2/g左右,只比不用模板剂的样品略有增加;而添加模板剂的样品孔容在0.6~0.83 mL/g之间,明显高于PEG-sol-0,原因可能是硅溶胶中大部分SiO2为10∼20 nm的颗粒,PEG分子链无法进入其内部并与之高度均匀混合,因此不能起到“撑起”孔道的作用,即PEG分子只能存在于SiO2粒子间的孔隙内,当凝胶烘干和灼烧时PEG分子气化散失,只能为SiO2材料留下较大的粒间孔,对比表面积的贡献很小,所以样品的比表面积没有明显变化,只是孔容明显增加. 因此可以推断,只有当硅源中的硅前体从分子或多分子状态开始凝胶化过程时(如PTEOS),简单有机模板才能起到提高比表面积和孔容的作用;当硅前体是SiO2颗粒时(如硅溶胶),简单有机模板不能进入其内部,所以得不到高比表面积的SiO2产物.表3 以硅溶胶为硅源所得多孔SiO2样品的比表面和累积孔容Table 3 BET surface area and pore structure results of poroussilica with silica sol as silicon sourceSampleS BET(m2/g)V meso1)(mL/g)V BJH2)(mL/g)Averagepore size (nm)PEG2000-sol-0 201 0.11 0.19 3.78PEG2000-sol-0.1 262 0.54 0.62 9.46 PEG2000-sol-0.3 249 0.58 0.65 10.4 PEG2000-sol-0.5 248 0.74 0.83 13.4 Note: 1) Pore size 1.5∼50 nm; 2) Pore size 1.5∼200 nm.4 结 论(1) 采用TEOS为硅源,PEG和PVP、松香为简单有机模板,制备了比表面积大于650 m2/g的多孔SiO2,其中分子量为2000的PEG(PEG2000)是较好的模板剂,所制SiO2样品孔容大于1 mL/g,其孔道多在介孔范围,属无规则孔道.(2) 根据实验结果提出了简单有机模板可能的致孔机理:TEOS聚合物首先与模板剂发生分子尺度的均匀混合,在快速凝胶过程中产生了纳米尺度杂化的有机−无机复合材料,在干燥和灼烧过程中有机物挥发散失,从而赋予材料发达的孔隙结构和较高的比表面积. 采用碱性硅溶胶为硅源进行了对比实验,发现简单有机模板不能明显提高样品的比表面积,原因在于硅溶胶以粒度10∼20 nm的胶粒为凝胶基本单位,有机模板无法进入其内部起到“撑起”孔道的作用,这也一定程度上证实了上述多孔SiO2形成机理的正确性.(3) 该方法可方便快捷地得到具有无规则孔道的高比表面积SiO2材料.参考文献:[1] Kresge C T, Leoniwicz M E, Roth W J. Ordered MesoporousMolecular Sieves Synthesized by a Liquid-crystal Template Mechanism [J]. Nature, 1992, 359: 710−712.[2] Beck J S, Vartuli J C, Roth W J. A New Family of MesoporousMolecular Sieves Prepared with Liquid Crystal Templates [J]. J. Am.Chem. Soc., 1992, 114: 10834−10843.[3] Huo Q, Leon R, Petroff P M. Mesostructure Design with GeminiSurfactants: Supercage Formation in a Three-dimensional Hexagonal Array [J]. Science, 1995, 268: 1324−1327.[4] Huo Q, Margolese D I, Stucy G D. Surfactant Control of Phases in theSynthesis of Mesoporous Silica-based Materials [J]. Chem. Mater., 1996, 8: 1147−1160.[5] Tanev P T, Pinnavaia T J. A Neutral Templating Route to MesoporousMolecular Sieves [J]. Science, 1995, 267: 865−867.[6] Tanev P T, Pinnavaia T J. Mesoporous Silica Molecular SievesPrepared by Ionic and Neutral Surfactant Templating: A Comparison of Physical Properties [J]. Chem. Mater., 1996, 8: 2068−2079.[7] Tanev P T, Pinnavaia T J. Biomimetic Templating of Porous LamellarSilicas by Vesicular Surfactant Assemblies [J]. Science, 1996, 271: 1267−1269.[8] Zhao D, Huo Q, Feng J. Tri-, Tetra- and Octablock Copolymer andNonionic Surfactant Syntheses of Highly Ordered, Hydrothermally Stable, Mesoprous Silica Structures [J]. J. Am. Chem. Soc., 1998, 120: 6024−6036.[9] Zhao D, Feng J, Huo Q. Triblock Copolymer Syntheses ofMesoporous Silica with Periodic 50 to 300 Angstrom Pores [J].Science, 1998, 279: 548−552.[10] Soleimani Dorcheh A, Abbasi M H. Silica Aerogel, Synthesis,Properties and Characterization [J]. J. Mater. Process. Technol., 2008, 199: 10–26.[11] Wu Y H, Wu C M, Yu F, et al. Free-standing Anion-exchangePEO–SiO2 Hybrid Membranes [J]. J. Membr. Sci., 2008, 307: 28–36.1010 过程工程学报第9卷[12] Chang K Ch, Chen Y K, Chen H. Preparation and Characterizationof Superhydrophobic Silica-based Surfaces by Using Polypropylene Glycol and Tetraethoxysilane Precursors [J]. Surf. Coat. Technol., 2007, 201: 9579–9586.[13] Haruko S, Kazuyoshi K, Kazuki N, et al. Three-dimensionalObservation of Macroporous Silica Gels and the Study on Structural Formation Mechanism [J]. Colloids Surf., A, 2007, 300: 245–252. [14] Gallardo J, Galliano P G, Porto Lopez J M. Preparation and in vitroEvaluation of Porous Silica Gels [J]. Biomaterials, 2002, 23: 4277–4284.[15] Martin J, Hosticka B, Lattimer C, et al. Mechanical and AcousticalProperties as a Function of PEG Concentration in Macroporous Silica Gels [J]. J. Non-Cryst. Solids, 2001, 285: 222−229. [16] 王娟,张长瑞,冯坚. 聚乙二醇对纳米多孔二氧化硅薄膜性能的影响 [J]. 无机材料学报, 2005, 20(2): 436−441.[17] 谷景华,吴波玲,张跃. 多孔二氧化硅块材的制备 [J]. 稀有金属材料与工程,2008, 36(Suppl.1): 545−548.[18] 何飞,郝晓东,李垚. 二氧化硅干凝胶的制备与结构分析 [J]. 材料工程, 2005, (12): 13−16.[19] 沈军,周斌,吴广明,等. 纳米孔超级绝热材料气凝胶的制备与热学特性 [J]. 过程工程学报, 2002, 2(4): 341−345.[20] 孙继红,巩雁军,范文浩,等. SiO2−PEG凝胶体系织构特性的研究 [J]. 高等学校化学学报, 2000, 21(1): 95−98.[21] Kamiya K, Yoko T. Synthesis of SiO2 Glass Fibers fromSi(OC2H5)4−H2O−C2H5OH−HCl Solution through Sol−Gel Method [J]. J. Mater. Sci., 1986, 21(3): 842−848Preparation of Porous Silica with Simple Organic TemplateLIU Hai-di, YUE Ren-liang, ZHANG Dong-hai, WU Zhen-jiang, CHEN Yun-fa (State Key Laboratory of Multi-phase Complex Systern, Institute of Process Engineering, CAS, Beijing 100190, China) Abstract: Porous silica was prepared with simple organic template and tetraethyl-orthosilicate (TEOS) as pore-generating agent and silicon precursor respectively. The influences of dosage of simple organic template upon the specific surface area and pore volume of the as-made porous silica were investigated. BET, SEM and TEM methods were employed to analyze the specific surface area and pore structure of the silica samples. A proposed mechanism was developed to explain the pore-forming effect of the simple organic template on the porous silica. Parallel experiments with base-silica-sol used as silicon precursor were also conducted to testify the proposed mechanism. The results indicated that when the dosage of the template was 0.3 g/mL TEOS, the samples with large surface area (>650 m2/g) and high pore volume (>1.0 mL/g) could be successfully prepared, and the pore structure was amorphous. It is highly possible that the amorphous pores are generated by the simple organic template which was mixed with TEOS polymer in molecular scale and was burnt away in the following calcination process.Key words: porous silica; sol−gel; template。
- 1、下载文档前请自行甄别文档内容的完整性,平台不提供额外的编辑、内容补充、找答案等附加服务。
- 2、"仅部分预览"的文档,不可在线预览部分如存在完整性等问题,可反馈申请退款(可完整预览的文档不适用该条件!)。
- 3、如文档侵犯您的权益,请联系客服反馈,我们会尽快为您处理(人工客服工作时间:9:00-18:30)。
文章编号:1001-2486(2005)02-0011-04常压干燥制备疏水SiO 2气凝胶!陈一民,谢凯,洪晓斌,肖加余(国防科技大学航天与材料工程学院,湖南长沙410073)摘要:以聚二乙氧基硅氧烷(PDEOS )为原料,用甲基三乙氧基硅烷(MTES )对制得的醇凝胶进行表面改性,经乙醇和正己烷洗涤,在常压条件下干燥后得到疏水二氧化硅气凝胶。
研究表明:溶胶—凝胶体系中各组分的最佳配比为C 2H 5OH=PDEOS=HF=H 2O =20=10=3=0.5(重量比);当MTES /PDEOS (重量比)大于1.2时,经表面改性的疏水二氧化硅气凝胶与水的接触角大于110 ,其密度和比表面积分别在125~160kg /m 3和560~900m 2/g 范围。
关键词:二氧化硅;气凝胶;疏水;常压干燥中图分类号:O648文献标识码:APreparation of Hydrophobic SiO 2Aerogels at Ambient PressureCHEN Yi-min ,XIE Kai ,HONG Xiao-bin ,XIAO Jia-yu(CoIIege of Aerospace and MateriaI Engineering ,NationaI Univ.of Defense TechnoIogy ,Changsha 410073,China )Abstract :The aIcogeI prepared by soI-geI process of poIydiethoxysiIoxane (PDEOS )according to the mass ratio of C 2H 5OH =PDEOS=HF=H 2O =20=10=3=0.5,was modified by methyItriethoxysiIane(MTES )and washed with aIcohoI and n-hexane.The obtained geI was then dried under ambient pressure to prepare hydrophobic SiO 2aerogeI.It is shown that hydrophobic SiO 2aerogeIs can be obtained when the mass ratio of MTES /PDEOS is higher than 1.2.The contact angIe between hydrophobic SiO 2aerogeIs and water is Iarger than 110 ,the densities and specific surface areas of hydrophobic SiO 2aerogeIs are in the range of 125~160kg /m 3and 560~900m 2/g respectiveIy.Key words :siIicon dioxide ;aerogeI ;hydrophobic ;ambient drying二氧化硅气凝胶在作为催化剂载体和隔热材料方面的应用展现了诱人前景。
但是,由于二氧化硅气凝胶制备采用昂贵的有机先驱体(正硅酸乙酯或正硅酸甲酯)作为硅源制备醇凝胶,并在超临界干燥条件下除去醇凝胶中的溶剂,而超临界干燥需要在高温高压或高压下进行(乙醇的临界温度243.0C ,临界压力6.3MPa ;二氧化碳的临界温度31.1C ,临界压力7.29MPa ),对干燥容器的要求很高,大大增高了气凝胶的成本,同时操作也存在很大的危险性,欧洲曾发生气凝胶干燥高压装置爆炸的悲剧。
气凝胶制备的高成本和危险性阻碍了气凝胶的工业化生产[1,2]。
由于超临界干燥存在的问题,替代在高温高压或高压下的超临界干燥制备二氧化硅气凝胶工艺成为国内外研究的重点。
常压干燥不需要高压干燥设备,气凝胶的制备条件温和,有利于气凝胶进行工业化大规模生产[3-4]。
1实验部分1.1试剂聚二乙氧基硅氧烷,工业品;无水乙醇,分析纯;HF(40wt%),分析纯;甲基三乙氧基硅烷,化学纯。
!收稿日期:2004-10-09基金项目:航天支撑技术基金资助项目(2002-HT -GFKD -006);国防科技大学校预研资助项目作者简介:陈一民(1963—),男,副教授,博士。
国防科技大学学报第27卷第2期JOURNAL OF NA TIONAL UNIVERSITY OF DEFENSE TECHNOLOGY VoI.27No.22005!."醇凝胶的制备按一定的配比将聚二乙氧基硅氧烷与乙醇于烧杯中混合,在磁力搅拌器的搅拌下往烧杯中慢慢加入水和催化剂。
继续搅拌2min ,使反应物混合均匀。
将混合液倒入试管密封,然后置于60C 水浴中。
待凝胶形成后(将试管倾斜45 ,试管内液体不发生明显的流动,说明已凝胶),加入一定量的无水乙醇继续老化,每隔241换一次无水乙醇,老化总时间为4天。
!.#醇凝胶的改性、洗涤和干燥将改性剂(甲基三乙氧基硅烷)的正己烷溶液加入含凝胶的试管并密封,将试管放入60C 水浴中放置721进行表面改性。
改性完毕,倒出改性剂溶液,用正己烷洗涤凝胶,直至正己烷中不含改性剂。
最后,将凝胶放在60C 下干燥481,得到二氧化硅气凝胶。
!.$气凝胶的表征用JEOL JSM -5600LV 型扫描电子显微镜观察二氧化硅的外貌;用Nexus670傅立叶变换红外光谱仪研究表面改性反应的情况;用NDVAl000型孔径分布测试仪测定气凝胶的比表面积等结构参数;用改进的液体静力天平法测定气凝胶的密度;通过测出气凝胶表面水珠的润湿角表征气凝胶疏水性强弱[6]。
"结果与讨论".!溶胶—凝胶过程PDEOS(A )与正硅酸乙酯的预聚物结构相似,相当于正硅酸乙酯已进行了多次水解和缩合,因此以PDEOS 作为原料时,形成凝胶所需的水解程度较以正硅酸乙为原料时低,所需的水量要少。
PDEOS 的水解过程为:[Si -O ]9C 2H 5O OC 2H 5OC 2H 5+2OH 2O OC 2H !5[Si -O ]9HO OH OH +2OC 2H 5OH OH (A )(B )水解产物(B )缩聚形成三维网络结构的凝胶。
在PDEOS 体系的溶胶—凝胶过程中,溶剂乙醇用量、水量和催化剂对溶胶—凝胶过程有明显的影响。
2.l.l 乙醇用量对凝胶时间的影响乙醇不仅是反应体系的溶剂,同时还是PDEOS 水解反应的产物。
在其它反应物配比固定为PDEOS1HF1H2O =l01310.5(重量比)时,乙醇的用量对凝胶时间的关系如表l 所示。
表l乙醇用量与凝胶时间的关系Tab.l Reiation between aico1oi and geiation timeC 2H 5OH1PDEOS (mass ratio )l.l2.0 2.9 4.l 4.9Geiation time(min )8.l l2.5l4.9l7.620.7由表l 可知,随着乙醇用量的增加,凝胶时间不断延长。
这是因为随着溶剂用量的增加,水解和缩聚反应的反应物浓度都减小,降低了水解和缩聚速度;同时也使单位体积内由缩聚反应形成的小粒子簇数目变少,因而需要更多的相互交联才能形成充满整个容器的大粒子簇,两者共同作用导致凝胶时间变长。
所以,随乙醇用量的增加,凝胶时间逐渐增大。
2.l.2水量对凝胶时间的影响在PDEOS 的水解—缩聚过程中,水是水解反应的反应物,同时也是缩聚反应的产物。
水的用量对凝胶时间有着重要的影响。
实验中,C 2H 5OH1PDEOS1HF =201l013(重量比),改变水的用量,得到如表2所示水量对凝胶时间的影响。
由表2看出,随着体系中水量的减少,凝胶时间延长。
这是由于随着水量减少,水解反应速率下降,硅酸浓度降低,硅酸间缩聚反应速率也下降,从而延长了凝胶时间。
2l 国防科技大学学报2005年第2期表2水量对凝胶时间的影响Tab.2Effect of water concentration on geiation timePDEOSI H2O(mass ratio)0.10.150.30.50.7Geiation time(min) 2.2 3.418.331.1672.1.3催化剂对凝胶时间的影响催化剂对凝胶时间也有重要影响,本文以氢氟酸(40wt%)作为催化剂,PDEOS I C2H5OHI H2O=10I20 I0.5(重量比),研究了催化剂对凝胶时间的影响,结果如表3所示。
表3催化剂用量对凝胶时间的影响Tab.3Effect of cataiyst concentration on geiation timeHFI PDEOS(mass ratio)0.0610.0750.1000.2500.300Geiation time(min)422817116由表3可知,随着催化剂用量的增加,凝胶时间下降,这是因为加入催化剂以后,加快了PDEOS的水解,生成硅酸加快,提高了缩聚反应速度,缩短了凝胶时间。
综上所述,乙醇、水、催化剂对凝胶时间有很大的影响,考虑以上因素对气凝胶的结构和性能等方面的影响,最终确定溶胶—凝胶体系的配比为C2H5OHI PDEOSI HFI H2O=20I10I3I0.5(重量比)。
!.!凝胶的表面形貌和疏水性为了在常压下干燥制备气凝胶,必须对凝胶进行表面改性,使凝胶表面的羟基被惰性基团取代并得到疏水表面,从而避免干燥时凝胶孔表面羟基之间的相互缩合引起的不可逆收缩及凝胶结构的破坏,同时使凝胶骨架表面具有一定的憎水性,骨架和溶剂之间的接触角增大,大幅度地减少毛细管的附加压力[3,4]。
改性后得到的二氧化硅气凝胶(KD-4)的红外光谱如图1所示。
图1改性后二氧化硅气凝胶的红外光谱Fig.1FTIR spectrum of modified siiica aerogeis图2疏水气凝胶表面的水珠Fig.2Water of hydrophobic siiica aerogei surface从图1可以看出,在2975cm-1,1392cm-1,850cm-1附近出现了峰,说明二氧化硅气凝胶在表面改性后,气凝胶骨架表面接上了硅甲基;在1088cm-1,461cm-1附近出现的峰分别代表Si-O-Si的反对称伸缩振动和弯曲振动。
在960cm-1附近存在的峰代表Si-OH伸缩振动的峰基本不明显,这说明二氧化硅气凝胶在经MTES表面疏水改性后硅羟基非常少。
图2是经表面改性的疏水气凝胶(KD-4)表面的水珠,接触角(!)约130 ,表明改性后的气凝胶表面疏水性很强。