Exfoliation of graphite oxide in propylene carbonate and thermal reduction附件
石墨烯的制备及其在铅酸电池中的应用
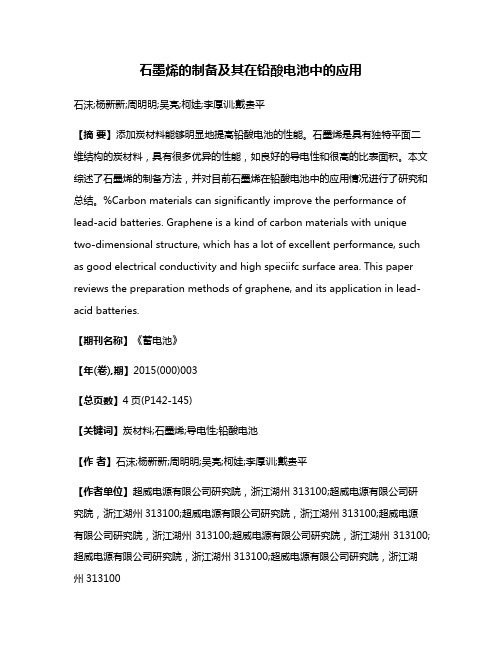
石墨烯的制备及其在铅酸电池中的应用石沫;杨新新;周明明;吴亮;柯娃;李厚训;戴贵平【摘要】添加炭材料能够明显地提高铅酸电池的性能。
石墨烯是具有独特平面二维结构的炭材料,具有很多优异的性能,如良好的导电性和很高的比表面积。
本文综述了石墨烯的制备方法,并对目前石墨烯在铅酸电池中的应用情况进行了研究和总结。
%Carbon materials can significantly improve the performance of lead-acid batteries. Graphene is a kind of carbon materials with unique two-dimensional structure, which has a lot of excellent performance, such as good electrical conductivity and high speciifc surface area. This paper reviews the preparation methods of graphene, and its application in lead-acid batteries.【期刊名称】《蓄电池》【年(卷),期】2015(000)003【总页数】4页(P142-145)【关键词】炭材料;石墨烯;导电性;铅酸电池【作者】石沫;杨新新;周明明;吴亮;柯娃;李厚训;戴贵平【作者单位】超威电源有限公司研究院,浙江湖州313100;超威电源有限公司研究院,浙江湖州313100;超威电源有限公司研究院,浙江湖州313100;超威电源有限公司研究院,浙江湖州313100;超威电源有限公司研究院,浙江湖州313100;超威电源有限公司研究院,浙江湖州313100;超威电源有限公司研究院,浙江湖州313100【正文语种】中文【中图分类】TM912.1石墨烯是碳原子紧密堆积的二维蜂窝状晶格结构的碳质材料,碳原子排列呈平面六边形结构,在二维平面上每个碳原子以 sp2杂化轨道相连接[1]。
氧化石墨烯的制备及表征
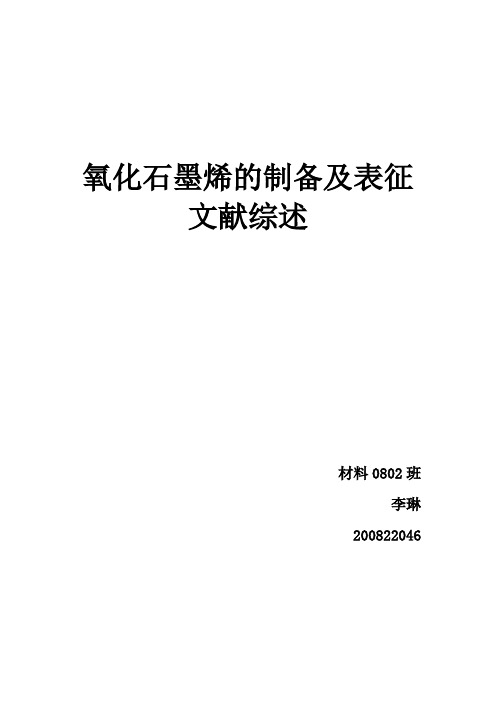
氧化石墨烯的制备及表征文献综述材料0802班李琳200822046氧化石墨烯的制备及表征李琳摘要:石墨烯(又称单层石墨或二维石墨)是单原子厚度的二维碳原子晶体,被认为是富勒烯、碳纳米管和石墨的基本结构单元[1]。
石墨烯可通过膨胀石墨经过超声剥离或球磨处理来制备[2,3],其片层厚度一般只能达到30~100 nm,难以得到单层石墨烯(约0.34 nm),并且不容易重复操作。
所以寻求一种新的、容易和可以重复操作的实验方法是目前石墨烯研究的热点。
而将石墨氧化变成氧化石墨,再在超声条件下容易得到单层的氧化石墨溶液,再通过化学还原获得,已成为石墨烯制备的有效途径[4]。
通过述评氧化石墨及氧化石墨烯的制备、结构、改性及其与聚合物的复合,展望了石墨烯及其复合材料的研究前景。
关键词:氧化石墨烯,石墨烯,氧化石墨,制备,表征Oxidation of graphite surfaces preparation and CharacterizationLI LinAbstrat:Graphite surfaces (also called single graphite or 2 d graphite )is the single atoms thickness of the 2 d carbon atoms crystal, is considered fullerenes, carbon nanotubes and graphite basic structure unit [1].Graphite surfaces can through the expanded graphite after ultrasonic stripping or ball mill treatment topreparation [2,3], a piece of layer thickness normally only up to 30 to 100 nm, hard to get the single graphite surfaces (about 0.34 nm), and not easy to repeated operation. So to search a new, easy to operate and can be repeated the experiment method of the graphite surfaces is the focus of research. And will graphite oxidization into oxidation graphite, again in ultrasonic conditions to get the oxidation of the single graphite solution, again through chemical reduction get, has become an effective way of the preparation of graphite surfaces [4]. Through the review of graphite oxide and oxidation graphite surfaces of the preparation, structure, modification of polymer and thecompound, and prospects the graphite surfaces and the research prospect of composite materials.Key words:Oxidation graphite surfaces, graphite surfaces, oxidation graphite, preparation,characterization采用Hummers 方法[5]制备氧化石墨。
X-ray photoelectron spectroscopy of reduced graphene oxide
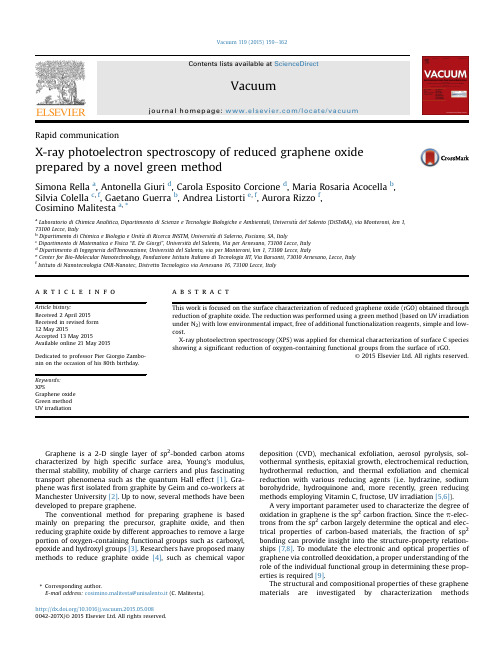
Rapid communicationX-ray photoelectron spectroscopy of reduced graphene oxide prepared by a novel green methodSimona Rella a ,Antonella Giuri d ,Carola Esposito Corcione d ,Maria Rosaria Acocella b ,Silvia Colella c ,f ,Gaetano Guerra b ,Andrea Listorti e ,f ,Aurora Rizzo f ,Cosimino Malitesta a ,*aLaboratorio di Chimica Analitica,Dipartimento di Scienze e Tecnologie Biologiche e Ambientali,Universit adel Salento (DiSTeBA),via Monteroni,km 1,73100Lecce,Italy b Dipartimento di Chimica e Biologia e Unit a di Ricerca INSTM,Universit a di Salerno,Fisciano,SA,Italy c Dipartimento di Matematica e Fisica “E.De Giorgi ”,Universit a del Salento,Via per Arnesano,73100Lecce,Italy d Dipartimento di Ingegneria dell'Innovazione,Universit a del Salento,via per Monteroni,km 1,73100Lecce,Italy eCenter for Bio-Molecular Nanotechnology,Fondazione Istituto Italiano di Tecnologia IIT,Via Barsanti,73010Arnesano,Lecce,Italy fIstituto di Nanotecnologia CNR-Nanotec,Distretto Tecnologico via Arnesano 16,73100Lecce,Italya r t i c l e i n f oArticle history:Received 2April 2015Received in revised form 12May 2015Accepted 13May 2015Available online 21May 2015Dedicated to professor Pier Giorgio Zambo-nin on the occasion of his 80th birthday.Keywords:XPSGraphene oxide Green method UV irradiationa b s t r a c tThis work is focused on the surface characterization of reduced graphene oxide (rGO)obtained through reduction of graphite oxide.The reduction was performed using a green method (based on UV irradiation under N 2)with low environmental impact,free of additional functionalization reagents,simple and low-cost.X-ray photoelectron spectroscopy (XPS)was applied for chemical characterization of surface C species showing a signi ficant reduction of oxygen-containing functional groups from the surface of rGO.©2015Elsevier Ltd.All rights reserved.Graphene is a 2-D single layer of sp 2-bonded carbon atoms characterized by high speci fic surface area,Young's modulus,thermal stability,mobility of charge carriers and plus fascinating transport phenomena such as the quantum Hall effect [1].Gra-phene was first isolated from graphite by Geim and co-workers at Manchester University [2].Up to now,several methods have been developed to prepare graphene.The conventional method for preparing graphene is based mainly on preparing the precursor,graphite oxide,and then reducing graphite oxide by different approaches to remove a large portion of oxygen-containing functional groups such as carboxyl,epoxide and hydroxyl groups [3].Researchers have proposed many methods to reduce graphite oxide [4],such as chemical vapordeposition (CVD),mechanical exfoliation,aerosol pyrolysis,sol-vothermal synthesis,epitaxial growth,electrochemical reduction,hydrothermal reduction,and thermal exfoliation and chemical reduction with various reducing agents (i.e.hydrazine,sodium borohydride,hydroquinone and,more recently,green reducing methods employing Vitamin C,fructose,UV irradiation [5,6]).A very important parameter used to characterize the degree of oxidation in graphene is the sp 2carbon fraction.Since the p -elec-trons from the sp 2carbon largely determine the optical and elec-trical properties of carbon-based materials,the fraction of sp 2bonding can provide insight into the structure-property relation-ships [7,8].To modulate the electronic and optical properties of graphene via controlled deoxidation,a proper understanding of the role of the individual functional group in determining these prop-erties is required [9].The structural and compositional properties of these graphene materials are investigated by characterization methods*Corresponding author.E-mail address:cosimino.malitesta@unisalento.it (C.Malitesta).Contents lists available at ScienceDirectVacuumjournal ho mep age:/lo cate/vacuum/10.1016/j.vacuum.2015.05.0080042-207X/©2015Elsevier Ltd.All rights reserved.Vacuum 119(2015)159e 162commonly applied in materials science.These include,for example,X-ray diffraction(XRD),X-ray photoelectron spectros-copy(XPS),transmission electron microscopy(TEM),scanning electron microscopy(SEM),atomic force microscopy(AFM),op-tical microscopy,Raman spectroscopy,and Fourier transform infrared spectroscopy(FTIR).The high specific area of the material has promoted an extensive use of XPS in its characterization(see e.g.Refs.[3,5,6,10e18])also with the aim to evidence(surface)contamination originating from preparation process and possibly resulting in considerable negative effects on electronic and optical properties.Previous studies reported the use of XPS in the characterization of reduced graphene oxide.In particular,a detailed analysis of C1s core level spectra allowed to reveal the content and nature of car-bon bonds attached to oxygen groups(C sp2,C sp3,C e OH e hy-droxyl,C e O e C e epoxy,>C e O e carbonyl,COOH e carboxyl,C(O) OC e ester,etc.).In this work,XPS is used to investigate the reduction of graphite oxide(GO)by a novel green method which conjugates the appli-cation of UV irradiation of an aqueous dispersion of GO[5]with the use of an inert atmosphere(made of N2),which proved to favor GO reduction[8,19].A scheme of photoreduction of graphite oxide is shown in Fig.1.The mechanism of UV photoreduction of graphite oxide has been the subject of several papers(see e.g.ref.[20]).GO samples were prepared by Hummers'oxidation method [21],starting from a high-surface-area graphite,HSAG.120mL of concentrated sulfuric acid and2.5g of sodium nitrate were intro-duced into a2000mL three-neck round bottomedflask immersed into an ice bath and5g of graphite were added,under nitrogen, with magnetic stirring.After obtaining a uniform dispersion of graphite powders,15g of potassium permanganate were added very slowly to minimize the risk of explosion.The reaction mixture was thus heated to35 C and stirred for24h.The resulting dark green slurry wasfirst poured into a copious amount of deionized water,and then centrifuged at10,000rpm for15min with a Hermle Z323K centrifuge.The isolated GO powder wasfirst washed twice with100mL of a5wt.%HCl aqueous solution and subsequently extensively washed with deionized water.Finally,it was dried at 60 C for24h.Further information about GO are reported in pre-vious papers[21,22].GO was dispersed in water(0.1mg/mL)by magnetic stirring for 30min followed by sonication for1h.Few milliliters of GO/water dispersion were degased by N2and placed into a UV oven(3D systems,50/60Hz)for48h under a nitrogen atmosphere.Reduced graphene oxide(rGO)undergoes to complete exfoliation,as proved by XRD data(not reported),resulting in a graphene material.For XPS measurements,the samples were dried and anchored by copper adhesive tape to the bar of the unit sample holder and then introduced into the analysis chamber through a transfer probe.X-ray photoelectron spectroscopy(XPS)measurements of graphite oxide(GO)and reduced graphene oxide(rGO)were per-formed with a Kratos Axis Ultra electron spectrometer using monochromatic Al K a source(1486.6eV)operated at225W(15kV, 15mA).Instrumental resolution(FWHM)at a pass energy of20eV as evaluated on the Ag3d5/2was0.60eV and on standard PET is 1.0eV.Survey scan spectra were recorded using pass energy of160eV and a1eV step.Narrow scans of C1s and O1s were acquired using a pass energy of20eV and a0.1eV step.The hybrid lens mode was used for all measurements.In each case the area of analysis was about700m m*300m m.During the acquisition a system of neutralization of the charge has been used.Base pressure in the analysis chamber was2.5*10À9torr.No degradation was observed under X-ray irradiation.Process-ing of the spectra,includingfitting of high resolution spectra,was accomplished with CasaXPS Release2.3.16software.The binding energy(BE)scale was referenced to the C1s line of graphitic car-bon/C sp2,set at284.50eV[23].Fitting of C1s signal was performed by subtracting Shirley background and using three components.A GL(30)lineshape(a combination of Gaussian70%and Lorentzian30%)was employed for a C e O component,including hydroxyl,epoxide,etc.,and a C]O component.In addition,a graphitic carbon/C sp2component was fitted with Voigt line shape exhibiting a higher binding energy tail [24].In particular,asymmetry was defined by a function LA(a,b,m), where a and b define the spread of the tail on either side of the Lorentzian component and the parameter m specifies the width of the Gaussian used to convolute the Lorentzian curve.While FWHM of GL components were free parameters infitting procedure,LA parameters and relevant FWHM of graphitic carbon/C sp2compo-nent werefixed.Assignment of peaks was based on literature[5,6,14,18].Fig.2(A)shows C1s XP spectrum of graphite oxide.It clearly indicates a considerable degree of oxidation in agreement with literature data[5,6,14,18].The high binding energy component could include a carboxylic contribute as it is wider than other components.After reduction,the peak for the graphitic carbon/C sp2component becomes largely dominant(see Fig.2(B)).Although the C1s XPS spectrum of reduced graphene oxide still exhibits ox-ygen functional groups,their intensities are much smaller than those in graphite oxide(Fig.2).This shows that most of oxygen-containing functional groups were removed upon thereduction.Fig.1.Scheme of photoreduction of graphite oxide to produce reduced graphene oxide.Irradiation results also in exfoliation of graphite matrix.S.Rella et al./Vacuum119(2015)159e162160The relative concentrations of surface functional groups ob-tained from fitting of the C 1s XPS regions are summarized in Table 1,along with fitting parameters.An evaluation of oxidation degree of analyzed materials can be obtained by rationing the area of graphitic carbon/C sp 2component and the sum of areas of oxygenated components (Table 2).It is evident the signi ficant removal of oxygenated groups upon reduction.In particular,as expected,reduction proved ef ficient in N 2atmosphere.Table 3compares our results with findings reported in other graphite oxide reduction studies.In comparison to previous methods (Table 3),the proposed one (much more ef ficient than methods Nos.2e 6,8e 10,12)is quite comparable in ef ficiency to those employing reductants,in solution such as hydrazine and NaBH 4(see Nos.11and 13),and plasma assisted reduction (see No.7).However,the present approach seems superior because it is low cost,easy to apply and has a low environmental impact.In fact,it not required the use of toxic reducing agents or complex and expensive instrumentation (like plasma reactor).This improvement can facilitate the large-scale production of graphene from graphite oxide for a number of applications.In conclusion,we successfully prepared reduced graphene oxide with a green method,as proved by XPS analysis.The proposed method appears better than previous ones in term of degree of reduction or of easy application.The realization of counter elec-trodes in composite polymeric material containing this graphene for solar cells is under investigation.AcknowledgmentsThis work was financially supported by Caripuglia.References[1]Dreyer DR,Park S,Bielawski CW,Ruoff RS.The chemistry of graphene oxide.Chem Soc Rev 2010;39:228e 40./10.1039/b917103g .[2]Novoselov KS,Geim AK,Morozov SV,Jiang D,Zhang Y,Dubonos SV,et al.Electric field effect in atomically thin carbon films.Science 2004;306:666e 9./10.1126/science.1102896.[3]Hsiao M-C,Liao S-H,Yen M-Y,Teng C-C,Lee S-H,Pu N-W,et al.Preparationand properties of a graphene reinforced nanocomposite conducting plate.J Mater Chem 2010;20:8496./10.1039/c0jm01679a .[4]Teng C-C,Ma C-CM,Lu C-H,Yang S-Y,Lee S-H,Hsiao M-C,et al.Thermalconductivity and structure of non-covalent functionalized graphene/epoxy composites.Carbon N Y 2011;49:5107e 16./10.1016/j.carbon.2011.06.095.[5]Ding YH,Zhang P,Zhuo Q,Ren HM,Yang ZM,Jiang Y.A green approach to thesynthesis of reduced graphene oxide nanosheets under UVirradiation.Fig.2.Fitted C 1s XP spectrum of graphite oxide (A)and reduced graphene oxide (B)after UV reduction (48h in N 2atmosphere).Table 1Results of fitting of C 1s XPS regions of graphite oxide before and after treatment.AssignmentGO r-GOUV oven for 48h in N 2atmosphere BE [eV]Lineshape FWHM Surface group distribution [%]BE [eV]Lineshape FWHM Surface group distribution [%]C sp 2284.50LA(1.3,3,3) 1.0069.01284.50LA(1.3,3,3) 1.0083.76C e O 286.74GL(30) 1.1817.75286.54GL(30) 1.218.01C ]O288.19GL(30)2.2713.24288.34GL(30)2.078.23Table 2Degree of oxidation.RatioGOr-GOUV oven for 48h in N 2atmosphere(Graphitic component)/(oxygenated components)2.225.15Table 3Summary of C sp 2component/oxygenated components ratios of rGO prepared by different approaches.(*)estimated from Fig.6(b)of the Ref.No.[5].No.Reduction methodRatio Ref.1UV irradiation 48h in N 2atmosphere 5.15Present work 2UV irradiation1.39(*)[5]3Thermal reduction oven e 220 C e air2.13[18]4Solvothermal reduction H 2O e 100 C e 24h 0.90[25]5Solvothermal reduction DMF e 150 C e 1h 1.986Thermal annealing in Ar/H 2atmosphere [150 C]2.57[26]7Plasma-assisted reduction in Ar/H 2atmosphere [150 C] 5.028Microwave-irradiation process in hydrogen plasma3.75[27]9g -ray irradiation in alcohol/water4.21[28]10Reduction agent:NaBH 4e 1h 2.58[29]11Reduction agent:NaBH 4e 48h5.812Reduction agent:N 2H 4e 1h 2.7813Reduction agent:N 2H 4e 48h5.8S.Rella et al./Vacuum 119(2015)159e 162161Nanotechnology2011;22:215601./10.1088/0957-4484/22/ 21/215601.[6]Kuila T,Bose S,Khanra P,Mishra AK,Kim NH,Lee JH.A green approach for thereduction of graphene oxide by wild carrot root.Carbon N Y2012;50:914e21./10.1016/j.carbon.2011.09.053.[7]Eda G,Chhowalla M.Chemically derived graphene oxide:towards large-areathin-film electronics and optoelectronics.Adv Mater2010;22:2392e415./10.1002/adma.200903689.[8]Pei S,Cheng H-M.The reduction of graphene oxide.Carbon N Y2012;50:3210e28./10.1016/j.carbon.2011.11.010.[9]Johari P,Shenoy VB.Modulating optical properties of graphene oxide:role ofprominent functional groups.ACS Nano2011;5:7640e7./10.1021/nn202732t.[10]Speranza G,Minati L,Anderle M.The C1s core line in irradiated graphite.J Appl Phys2007;102:043504./10.1063/1.2769332.[11]Yang D,Velamakanni A,Bozoklu G,Park S,Stoller M,Piner RD,et al.Chemicalanalysis of graphene oxidefilms after heat and chemical treatments by X-ray photoelectron and Micro-Raman spectroscopy.Carbon N Y2009;47:145e52./10.1016/j.carbon.2008.09.045.[12]Estrade-Szwarckopf H.XPS photoemission in carbonaceous materials:a“defect”peak beside the graphitic asymmetric peak.Carbon N Y2004;42: 1713e21./10.1016/j.carbon.2004.03.005.[13]Perrozzi F,Prezioso S,Donarelli M,Bisti F,De Marco P,Santucci S,et e ofoptical contrast to estimate the degree of reduction of graphene oxide.J Phys Chem C2013;117:620e5./10.1021/jp3069738.[14]Paredes JI,Villar-Rodil S,Fern a ndez-Merino MJ,Guardia L,Martínez-Alonso A,Tasc o n JMD.Environmentally friendly approaches toward the mass produc-tion of processable graphene from graphite oxide.J Mater Chem2011;21:298./10.1039/c0jm01717e.[15]Mattevi C,Eda G,Agnoli S,Miller S,Mkhoyan KA,Celik O,et al.Evolution ofelectrical,chemical,and structural properties of transparent and conducting chemically derived graphene thinfilms.Adv Funct Mater2009;19:2577e83./10.1002/adfm.200900166.[16]Lesiak B,Stobinski L,Malolepszy A,Mazurkiewicz M,K€o v e r L,T o th J.Prepa-ration of graphene oxide and characterisation using electron spectroscopy.J Electron Spectrosc Relat Phenom2014;193:92e9./10.1016/ j.elspec.2014.03.015.[17]Stobinski L,Lesiak B,Malolepszy A,Mazurkiewicz M,Mierzwa B,Zemek J,et al.Graphene oxide and reduced graphene oxide studied by the XRD,TEMand electron spectroscopy methods.J Electron Spectrosc Relat Phenom 2014;195:145e54./10.1016/j.elspec.2014.07.003.[18]Zangmeister CD.Preparation and evaluation of graphite oxide reduced at220 C.Chem Mater2010;22:5625e9./10.1021/cm102005m.[19]Kundu S,Wang Y,Xia W,Muhler M.Thermal stability and reducibility ofoxygen-containing functional groups on multiwalled carbon nanotube sur-faces:a quantitative high-resolution XPS and TPD/TPR study.J Phys Chem C 2008;112:16869e78./10.1021/jp804413a.[20]Plotnikov VG,Smirnov VA,Alfimov MV,Shul'ga YM.The graphite oxidephotoreduction mechanism.High Energy Chem2011;45:411e5.http:// /10.1134/S0018143911050158.[21]Mauro M,Cipolletti V,Galimberti M,Longo P,Guerra G.Chemically reducedgraphite oxide with improved shape anisotropy.J Phys Chem C2012;116: 24809e13./10.1021/jp307112k.[22]Mauro M,Acocella MR,Corcione CE,Maffezzoli A,Guerra G.Catalytic activityof graphite-based nanofillers on cure reaction of epoxy resins.Polym(Guildf) 2014;55:5612e5./10.1016/j.polymer.2014.09.019.[23]Briggs D,Seah MP.Practical surface analysis,auger and X-ray photoelectronspectroscopy.2nd ed.vol.1990;1990.[24]Li X,Wang H,Robinson JT,Sanchez H,Diankov G,Dai H.Simultaneous ni-trogen doping and reduction of graphene oxide.J Am Chem Soc2009;131: 15939e44./10.1021/ja907098f.[25]Lin Z,Yao Y,Li Z,Liu Y,Li Z,Wong C-P.Solvent-assisted thermal reduction ofgraphite oxide.J Phys Chem C2010;114:14819e25./10.1021/jp1049843.[26]Lee SW,Mattevi C,Chhowalla M,Sankaran RM.Plasma-assisted reduction of gra-phene oxide at low temperature and atmospheric pressure forflexible conductor applications.J Phys Chem Lett2012;3:772e7./10.1021/jz300080p.[27]Eng AYS,Sofer Z, Simek P,Kosina J,Pumera M.Highly hydrogenated graphenethrough microwave exfoliation of graphite oxide in hydrogen plasma:to-wards electrochemical applications.Chemistry2013;19:15583e92.http:// /10.1002/chem.201303164.[28]Zhang B,Li L,Wang Z,Xie S,Zhang Y,Shen Y,et al.Radiation inducedreduction:an effective and clean route to synthesize functionalized graphene.J Mater Chem2012;22:7775./10.1039/c2jm16722k.[29]Shin H-J,Kim KK,Benayad A,Yoon S-M,Park HK,Jung I-S,et al.Efficientreduction of graphite oxide by sodium borohydride and its effect on electrical conductance.Adv Funct Mater2009;19:1987e92./10.1002/ adfm.200900167.S.Rella et al./Vacuum119(2015)159e162 162。
综述制备石墨烯的化学方法

The development of various methods for producing graphene — a single layer of carbon atoms bonded together in a hexagonal lattice — has stimulated a vast amount of research in recent years1. The remarkable properties of graphene reported so far include high values of its Young’s modulus (~1,100 GPa)2, fracture strength (125 GPa)2, thermal conductivity (~5,000 W m−1K−1)3, mobility of charge carriers (200,000 cm2 V−1 s−1)4 and specific surface area (calculated value, 2,630 m2 g−1)5, plus fascinating transport phenomena such as the quantum Hall effect6. Graphene and chemically modified graphene (CMG) are promising candidates as components in applications such as energy-storage materials5, ‘paper-like’ materials7,8, polymer composites9,10, liquid crystal devices11 and mechanical resonators12.
氧化石墨烯制备石墨烯的研究进展_庄亚芳
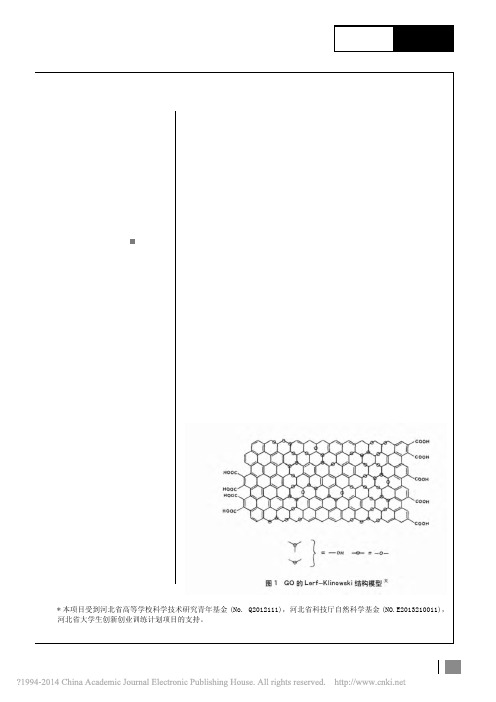
分散液。
在碱性环境中才能有效进行的局限。利 含氧基团脱去的速率很快,产生的压力
Li 等 [12] 在以往实验的基础上利用 聚乙二醇功能化 GO 的方法首次成功制
用该方法得到的石墨烯的 C/O 极高, 可达 12 以上,不仅能够做到不破坏石
能破坏石墨烯片层间的范德华力,从而 实 现 GO 的 剥 离。Tjong 等 [26] 发 现 当
2.3.2 固相微波法
固相微波法的使用也较为普遍,由
于微波加热过程中 GO 表面的含氧官能
团被分解成气体,产生的压强克服了片
层间的范德华力,所以 GO 得以成功剥
离。Hu 等 [36] 发 现 石 墨 烯 本 身 是 一 种
图 4 低温负压法制备的小批量 (a)以及宏量 (b)的石墨烯粉体材料 [29]
Thesis 论文选粹
氧
化
石
墨
石
烯
家 庄 庄铁
亚道
制 备
芳大
李 从 振
学 材 料 科
韩学
石 墨
刘与
春工
赵 永 克
程 学 院
︵
烯
河 北 石
的
家 庄
研
︶
究
进
展
【摘要】近年来,石墨烯已成为世界各国学者研究的热点。由石墨粉 先制备氧化石墨烯进而制备石墨烯成为了石墨烯最重要的制备方法。本文 综述了氧化石墨烯制备石墨烯的主要方法:化学法、热法、微波法、电化 学法、水热法、碱法等,并对各方法的优缺点进行了阐述,最后对石墨烯 的未来进行了展望。
在含有 H2 的 Ar 保护气中进行微波加 热,利用 H2 的还原作用促进了 GO 中 含 氧 官 能 团 的 还 原, 从 而 得 到 还 原 程
石墨烯表面活性剂

序号分散剂/活性剂的名称简介结构1N-甲基-吡咯化合物(烷酮)2十二烷基苯磺酸钠(SDBS)分子式:C18H29NaO3S;分子量:348.48;固体,白色或淡黄色粉末,易溶于水,易吸潮结块;无毒3聚乙烯吡咯烷酮(PVP)分子式:(C6H9NO)n;白色或乳白色粉末或颗粒;4阴离子型表面活性剂:木质素磺酸钠(SLS)多聚物;分子量不定;化学结构尚未确定5胆酸钠(SC)分子式:C24H39Nao5;分子量:430.55;白色结晶或无色粉末6高浓度的胆酸钠7十六烷基三甲基溴化铵(CTAB)分子式: C16H33(CH3)3NBr;分子量:364.446;白色微晶性粉末,吸湿性,在酸性溶液中稳定,易溶于乙醇8聚氧乙烯月桂醚(Brij 35)棕色粘稠液,易溶于水,具有乳化、润湿、分散能力标准情况下熔点约为27℃沸点约100℃密度接近于水约为1.00g/mL,闪点大于110℃折射率 1.462可以用作乳化润湿剂,在橡胶工业中用作分散剂,石油工业和环境保护行业中用作溢油分散剂的组分之一。
也可用作醚酯类非离子型表面活性剂9吐温80分子式:C64H124O26 ;分子量:1309.5 ;浅黄色粘稠液体10曲拉通X100聚乙二醇对异辛基苯基醚;分子式:C34H62O11;分子量:646.86;对人体有害。
11氧化二丁基锡分子式:C8H18OSn ,分子量:248.95 ,白色到微黄色粉末。
熔点 >300℃,水溶性 4.0 mg/L(20℃)。
溶于盐酸,不溶于水及有机溶剂。
遇火自燃;剧毒12Disperbyk-163分散剂 Disperbyk-163 是一种相对分子质量为 17000,的嵌段共聚物,包括亲颜料端的胺基和亲溶剂端的酯基和羧基。
13赖氨酸14聚间亚苯亚乙烯衍生物(PmPV)15DsPE16聚乙烯醇(PVA) 17聚丙烯酰胺购买/自行合成出现的文章结构/机理优缺点These solvents areexpensive and requirespecial care whenhandling可购买liquid phase production ofgraphene by exfoliation ofgraphite insurfactent/water solutions合成工艺成熟、成本价低,可以得到高品质的石墨烯,具有较广阔的应用前景,但是此方法制备的石墨烯浓度较低。
锂离子电池 英文
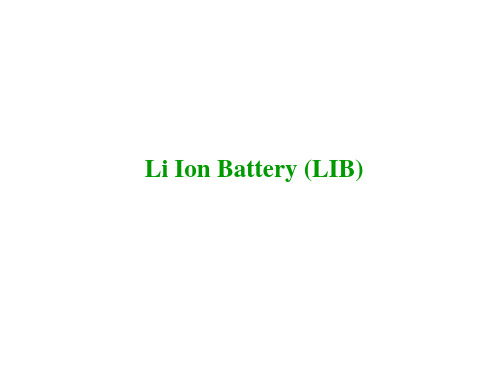
Electrolyte
• The selection of the electrolyte depends on the potential window. • Potentials are beyond water potential and hence non aqueous electrolyte. • It should not reactive with Li+ ions, since it will decide the transport of the Li+ ions for the intercalation reaction. • The electrolyte solution commonly comprises a lithium salt dissolved in a mixture of organic solvents. • Examples include LiPF6 or LiBOB (the BOB is the anion with the boron coordinated by two oxalate groups) in an ethylene carbonate/dimethyl carbonate solvent.
Li Ion Battery (LIB)
Battery
• Battery: Transducer which converts chemical energy into electrical energy. • Chemical reactions: Oxidation and reduction • Free energy change of the processes appears as electrical energy. • Primary battery-not rechargeable • Secondary battery- rechargeable
石墨烯的制备研究进展_袁小亚
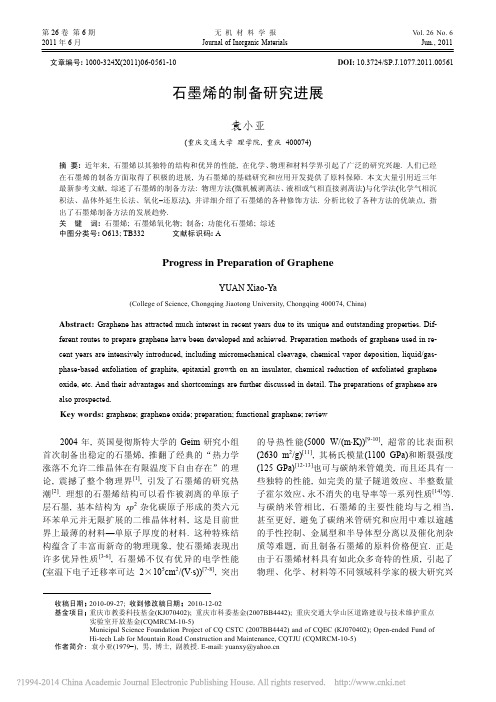
第26卷 第6期 无 机 材 料 学 报Vol. 26No. 62011年6月Journal of Inorganic Materials Jun., 2011收稿日期: 2010-09-27; 收到修改稿日期: 2010-12-02基金项目: 重庆市教委科技基金(KJ070402); 重庆市科委基金(2007BB4442); 重庆交通大学山区道路建设与技术维护重点实验室开放基金(CQMRCM-10-5)Municipal Science Foundation Project of CQ CSTC (2007BB4442) and of CQEC (KJ070402); Open-ended Fund ofHi-tech Lab for Mountain Road Construction and Maintenance, CQTJU (CQMRCM-10-5)作者简介: 袁小亚(1979−), 男, 博士, 副教授. E-mail: yuanxy@文章编号: 1000-324X(2011)06-0561-10 DOI: 10.3724/SP.J.1077.2011.00561石墨烯的制备研究进展袁小亚(重庆交通大学 理学院, 重庆 400074)摘 要: 近年来, 石墨烯以其独特的结构和优异的性能, 在化学、物理和材料学界引起了广泛的研究兴趣. 人们已经在石墨烯的制备方面取得了积极的进展, 为石墨烯的基础研究和应用开发提供了原料保障. 本文大量引用近三年最新参考文献, 综述了石墨烯的制备方法: 物理方法(微机械剥离法、液相或气相直接剥离法)与化学法(化学气相沉积法、晶体外延生长法、氧化−还原法), 并详细介绍了石墨烯的各种修饰方法. 分析比较了各种方法的优缺点, 指出了石墨烯制备方法的发展趋势.关 键 词: 石墨烯; 石墨烯氧化物; 制备; 功能化石墨烯; 综述中图分类号: O613; TB332 文献标识码: AProgress in Preparation of GrapheneYUAN Xiao-Ya(College of Science, Chongqing Jiaotong University, Chongqing 400074, China)Abstract: Graphene has attracted much interest in recent years due to its unique and outstanding properties. Dif-ferent routes to prepare graphene have been developed and achieved. Preparation methods of graphene used in re-cent years are intensively introduced, including micromechanical cleavage, chemical vapor deposition, liquid/gas- phase-based exfoliation of graphite, epitaxial growth on an insulator, chemical reduction of exfoliated graphene oxide, etc. And their advantages and shortcomings are further discussed in detail. The preparations of graphene are also prospected.Key words: graphene; graphene oxide; preparation; functional graphene; review2004年, 英国曼彻斯特大学的Geim 研究小组首次制备出稳定的石墨烯, 推翻了经典的“热力学涨落不允许二维晶体在有限温度下自由存在”的理论, 震撼了整个物理界[1], 引发了石墨烯的研究热潮[2]. 理想的石墨烯结构可以看作被剥离的单原子层石墨, 基本结构为sp 2杂化碳原子形成的类六元环苯单元并无限扩展的二维晶体材料, 这是目前世界上最薄的材料—单原子厚度的材料. 这种特殊结构蕴含了丰富而新奇的物理现象, 使石墨烯表现出许多优异性质[3-6], 石墨烯不仅有优异的电学性能(室温下电子迁移率可达2×105cm 2/(V·s))[7-8], 突出的导热性能(5000 W/(m·K))[9-10], 超常的比表面积(2630 m 2/g)[11], 其杨氏模量(1100 GPa)和断裂强度(125 GPa)[12-13]也可与碳纳米管媲美, 而且还具有一些独特的性能, 如完美的量子隧道效应、半整数量子霍尔效应、永不消失的电导率等一系列性质[14]等. 与碳纳米管相比, 石墨烯的主要性能均与之相当, 甚至更好, 避免了碳纳米管研究和应用中难以逾越的手性控制、金属型和半导体型分离以及催化剂杂质等难题, 而且制备石墨烯的原料价格便宜. 正是由于石墨烯材料具有如此众多奇特的性质, 引起了物理、化学、材料等不同领域科学家的极大研究兴562 无机材料学报第26卷趣, 也使得石墨烯在电子、信息、能源、材料和生物医药等领域具有重大的应用前景[3-6, 15].1石墨烯的制备方法概述目前有关石墨烯的制备方法, 国内外有较多的文献综述[4-6, 16-19], 石墨烯的制备主要有物理方法和化学方法. 物理方法通常是以廉价的石墨或膨胀石墨为原料, 通过微机械剥离法、液相或气相直接剥离法来制备单层或多层石墨烯, 此法原料易得, 操作相对简单, 合成的石墨烯的纯度高、缺陷较少, 但费时、产率低下, 不适于大规模生产. 目前实验室用石墨烯主要多用化学方法来制备, 该法最早以苯环或其它芳香体系为核, 通过多步偶联反应取代苯环或大芳香环上6个, 循环往复, 使芳香体系变大, 得到一定尺寸的平面结构的石墨烯(化学合成法)[20]. 2006年Stankovich等[21]首次用肼还原脱除石墨烯氧化物(graphene oxide, 以下简称GO)的含氧基团从而恢复单层石墨的有序结构(氧化−还原法), 在此基础上人们不断加以改进, 使得氧化−还原法(含氧化−修饰−还原法)成为最具有潜力和发展前途的合成石墨烯及其材料的方法[16]. 除此之外, 晶体外延生长、化学气相沉积也可用于大规模制备高纯度的石墨烯. 本文重点总结近三年化学法, 尤其是氧化−还原法制备石墨烯的研究进展, 并对制备石墨烯的各种途径的优缺点加以评述.2 物理法制备石墨烯2.1微机械剥离法微机械剥离法是最早用于制备石墨烯的物理方法. Geim等[1]在1mm厚的高定向热解石墨表面进行干法氧等离子刻蚀, 然后将其粘到玻璃衬底上, 接着在上面贴上1μm 厚湿的光刻胶, 经烘焙、反复粘撕, 撕下来粘在光刻胶上的石墨片放入丙酮溶液中洗去, 最后将剩余在玻璃衬底上的石墨放入丙醇中进行超声处理, 从而得到单层石墨烯. 虽然微机械剥离是一种简单的制备高质量石墨烯的方法, 但是它费时费力, 难以精确控制, 重复性较差, 也难以大规模制备.2.2液相或气相直接剥离法通常直接把石墨或膨胀石墨(EG)(一般通过快速升温至1000℃以上把表面含氧基团除去来获取)加在某种有机溶剂或水中, 借助超声波、加热或气流的作用制备一定浓度的单层或多层石墨烯溶液. Coleman等参照液相剥离碳纳米管的方式将石墨分散在N-甲基-吡咯烷酮 (NMP) 中, 超声1h后单层石墨烯的产率为1%[22], 而长时间的超声(462h)可使石墨烯浓度高达 1.2mg/mL, 单层石墨烯的产率也提高到4%[23]. 他们的研究表明[22], 当溶剂的表面能与石墨烯相匹配时, 溶剂与石墨烯之间的相互作用可以平衡剥离石墨烯所需的能量, 而能够较好地剥离石墨烯的溶剂表面张力范围为40~50mJ/m2; Hamilton等[24]把石墨直接分散在邻二氯苯(表面张力: 36.6mJ/m2)中, 超声、离心后制备了大块状(100~500nm)的单层石墨烯; Drzal等[25]利用液−液界面自组装在三氯甲烷中制备了表面高度疏水、高电导率和透明度较好的单层石墨烯. 为提高石墨烯的产率, 最近Hou等[26]发展了一种称为溶剂热插层(solvothermal-asssisted exfoliation)制备石墨烯的新方法(图1), 该法是以EG为原料, 利用强极性有机溶剂乙腈与石墨烯片的双偶极诱导作用(dipole- induced dipole interaction)来剥离、分散石墨, 使石墨烯的总产率提高到10%~12%. 同时, 为增加石墨烯溶液的稳定性, 人们往往在液相剥离石墨片层过程中加入一些稳定剂以防止石墨烯因片层间的范德华力而重新聚集. Coleman 研究小组在水/十二烷基苯磺酸钠( SDBS) 中超声处理石墨30min, 详细研究了石墨初始浓度以及SDBS浓度对石墨烯产率的影响, 发现所得的石墨烯多数在5层以下, 并且具有较高的导电率(~104 S/m)[27], 后来发现柠檬酸钠作为稳定剂也具有较好的剥离分散效果[28]. Englert 等[29]合成一种新型的水溶性含大芳香环的两亲性物质并作为片层石墨的稳定剂(图2), 利用该物质与石墨片层的π−π堆积与疏水作用来制备稳定的石墨烯水溶液. 最近, 为同时提高单层石墨烯的产率及其溶液的稳定性, Li等[30]提出“exfoliation-rein-tercalation-expansion”方法(图3), 以高温处理后图1 溶剂热剥离法制备石墨烯[26]Fig. 1 Schematic illustration of solvothermal-assisted exfo-liation and dispersion of graphene sheets in CAN[26](a) Pristine EG; (b) EG; (c) Insertion of CAN molecules into the inter-layers of EG; (d) Exfoliated graphene sheets dispersed in ACN; (e) Optical images of graphene solutions第6期袁小亚: 石墨烯的制备研究进展 563图2 合成的水溶性两亲性物质[29]Fig. 2 Soluble perylene-based bolaamphiphile detergent[29]图3 “剥离−再插层−膨胀”法制备石墨烯[30]Fig. 3 Route of “exfoliation-reintercalation-expansion” to graphene [30]的部分剥离石墨为原料, 用特丁基氢氧化铵插层后,再以DSPE-mPEG 为稳定剂, 合成的石墨烯90%为单层, 且透明度较高(83%~93%). 另外, 一些研究人员研究了利用气流的冲击作用来提高剥离石墨片层的效率, Janowska 等[31]以膨胀石墨为原料, 微波辐照下发现以氨水做溶剂能提高石墨烯的总产率(~8%), 深入研究证实高温下溶剂分解产生的氨气能渗入石墨片层中, 当气压超过一定数值足以克服石墨片层间的范德华力而使石墨剥离. Pu 等[32]将天然石墨浸入超临界CO 2中30min 以达到气体插层的目的, 经快速减压后将气体充入SDBS 的水溶液中即制得稳定的石墨烯水溶液, 该法操作简便、成本低, 但制备的石墨烯片层较多(~10层).因以廉价的石墨或膨胀石墨为原料, 制备过程不涉及化学变化, 液相或气相直接剥离法制备石墨烯具有成本低、操作简单、产品质量高等优点, 但也存在单层石墨烯产率不高、片层团聚严重、需进一步脱去稳定剂等缺陷. 为克服这种现象, 最近Knieke 等[33]发展了一种大规模制备石墨烯的方法, 即液相“机械剥离”. 该法采取了一种特殊的设备, 高速剪切含十二烷基磺酸钠的石墨水溶液, 3h 后溶液中单层和多层石墨烯的浓度高达25g/L, 而5h 后50%以上的石墨烯厚度小于3nm, 该法具有成本低、产率高、周期短等优势, 是一种极有诱惑力的大规模制备石墨烯的途径.3 化学法制备石墨烯3.1 化学气相沉积法(CVD)化学气相沉积(chemical vapor deposition, CVD) 是反应物质在相当高的温度、气态条件下发生化学反应, 生成的固态物质沉积在加热的固态基体表面, 进而制得固体材料的工艺技术. CVD 是工业上应用最广泛的一种大规模制备半导体薄膜材料的方法, 也是目前制备石墨烯的一条有效途径. Srivastava 等制备[34]采用微波增强CVD 在Ni 包裹的Si 衬底上生长出了约20nm 厚的花瓣状石墨片, 形貌并研究了微波功率对石墨片形貌的影响. 研究结果表明: 微波功率越大, 石墨片越小, 但密度更大. 此种方法制备的石墨片含有较多的Ni 元素. Zhu 等[35-36]用电感耦合射频等离子体CVD 在多种衬底上生长出纳米石墨微片. 这种纳米薄膜垂直生长在衬底上, 形貌类似于Srivastava 等[34]制备的“花瓣状”纳米片, 进一步研究发现这种方法生长出来的纳米石墨片平均厚度仅为1nm, 并且在透射电镜下观察到了垂直于衬底的单层石墨烯薄膜(厚0.335nm). Berger 等[37-38]将SiC 置于高真空(1.33×10−10 Pa)、1300 ℃下, 使SiC 薄膜中的Si 原子蒸发出来, 制备了厚度仅为1~2个碳原子层的二维石墨烯薄膜. 最近韩国成均馆大学研究人员[39]在硅衬底上添加一层非常薄的镍(厚度< 300nm), 然后在甲烷、氢气与氩气混合气流中加热至1000℃, 再将其快速冷却至室温, 即能在镍层上沉积出6~10层石墨烯, 通过此法制备的石墨烯电导率高、透明性好、电子迁移率高(~3700 cm 2 /(V·s)),并且具有室温半整数量子Hall 效应, 而且经图案化后的石墨烯薄膜可转移到不同的柔性衬底, 可用于制备大面积的电子器件(如电极、显示器等), 为石墨烯的商业化应用提供了一条有效的途径. CVD 法可满足规模化制备高质量、大面积石墨烯的要求, 但现阶段较高的成本、复杂的工艺以及精确的控制加工条件制约了CVD 法制备石墨烯的发展, 因此该法仍有待进一步研究[40-42].3.2 晶体外延生长法(SiC 高温退火)[43-44]通过加热单晶6H-SiC 脱除Si, 从而得到在SiC 表面外延的石墨烯. 将表面经过氧化或H 2刻蚀后的SiC 在高真空下通过电子轰击加热到1000℃以除掉表面的氧化物, 升温至1250~1450, ℃恒温1~20min, 可得到厚度由温度控制的石墨烯薄片. 这种方法得到的石墨烯有两种, 均受SiC 衬底的影响很大: 一564 无机材料学报第26卷种是生长在Si层上的石墨烯, 由于和Si层接触, 这种石墨烯的导电性受到较大影响, 一种生长在C层上的石墨烯则有着极为优良的导电能力. 这种方法条件苛刻(高温、高真空)、且制造的石墨烯不易以从衬底上分离出来, 难以能成为大量制造石墨烯的方法.3.3氧化−还原法(含氧化−修饰−还原法)这是目前最常用的制备石墨烯的方法, 国内外科学家已经对这方面做了大量的研究[16, 45-46]. 石墨本身是一种憎水性物质, 与其相比, GO表面和边缘拥有大量的羟基、羧基、环氧等基团, 是一种亲水性物质, 正是由于这些官能团使GO容易与其它试剂发生反应, 得到改性的氧化石墨烯; 同时GO层间距(0.7~1.2nm)[47]也较原始石墨的层间距(0.335nm)大, 有利于其它物质分子的插层. 制备GO的办法一般有3种: Standenmaier法[48]、Brodie法[49]、Hummers法[50]. 制备的基本原理均为先用强质子酸处理石墨, 形成石墨层间化合物, 然后加入强氧化剂对其进行氧化. 因这些方法中均使用了对化工设备有强腐蚀性、强氧化性的物质, 故现今有不少GO 的改进合成方法[51-52]. GO的结构比较复杂, 目前还没有公认的结构式, 比较常用的一种如图4所示[53] (关于GO化学结构的讨论可参阅[46, 54]).GO还原的方法包括化学液相还原[21]、热还原[55-56]、等离子体法还原[57]、氢电弧放电剥离[58]、超临界水还原[59]、光照还原[60-62]、溶剂热还原[63-64]、微波还原[65-68]等, 其中又以化学液相还原研究的最多, 常见的还原剂有水合肼[21, 69-72]、H2[73-74]、二甲肼[75]、对苯二酚[76]、NaBH4[77]、强碱[78]、MeReO3/ PPh3[51] 、纯肼[79]、Al粉[80]、维生素C[81-82]、乙二胺[83]、Na/CH3OH[84], Ruoff与Loh等对此作了很好的综述[46, 85]. 结构完整的二维石墨烯晶体表面呈惰性状态, 化学稳定性高, 与其它介质的相互作用较图4 石墨烯氧化物的结构式[53]Fig. 4 The structure of graphene oxide[53] 弱, 并且石墨烯片之间有较强的范德华力, 容易产生聚集, 使其难溶于水及常用的有机溶剂, 这给石墨烯的进一步研究和应用造成了很多困难. 为了充分发挥其优良性质、改善其可成型加工性(如提高溶解性、在基体中的分散性等), 必须对石墨烯表面进行有效的修饰, 通过引入特定的官能团, 还可以赋予石墨烯新的性质, 进一步拓展其应用领域. 修饰是实现石墨烯分散、溶解和成型加工的最重要手段[18]. 目前人们常采用先对GO进行修饰然后再进行还原(即氧化-修饰-还原). 其中, 石墨烯的修饰主要有共价键修饰和非共价键修饰[46, 85].3.3.1共价键修饰由于GO表面及边缘上有大量的羧基、羟基和环氧等活性基团, 可以充分利用这些官能团的活性进行多种化学反应(图5)在石墨烯片上引入各种分子即可达到石墨烯的共价键修饰.酰胺化反应是石墨烯共价修饰较常用的一个途径. 为增强COOH的反应活性, 通常先将其活化, 常用的活化试剂有二氯亚砜[86-90]、1-乙基-3-(3-二甲基胺丙基)−碳化二亚胺(EDC)[91]、N, N`-二环己基碳化二亚胺(DCC)[92-93]等. Niyogi等[86]先将GO上的羧基转变为酰氯(用SOCl2活化)然后与十八胺的胺基反应, 还原后制得长链烷基修饰的石墨烯在四氢呋喃(THF)的溶解度达0.5mg/mL, 且在四氯化碳、二氯甲烷等常用有机溶剂中也均有较好的溶解性. Bourlinos等也考察了各种伯胺、氨基酸与胺基硅氧烷共价修饰的石墨烯, 发现经修饰的石墨烯在水或有机溶液有极好的稳定性[94]. 除酰胺化反应外, COOH的酯化反应或其它反应也可用于修饰石墨烯. Shen等[95]将羧酸转变成其钠盐后然后利用亲核取代反应将正丁基引入石墨烯片上, 还原后发现经共价修饰的石墨烯在一些有机溶剂如氯仿、甲苯均有较好的稳定性, 且溶液的紫外−可见吸收光谱非常吻合朗伯−比尔定律. Salavagione等[90]采用核磁共振、红外光谱法等多种手段证实了聚乙烯醇(PV A)可成功通过酯化反应键合到石墨烯表面, 而Veca 等[92]则利用PV A侧链的羟基在GO表面的接枝制备PV A与石墨烯的复合物, 用作高分子合金的相容剂. Stankovich等[96]利用异氰酸酯与GO上的羧基和羟基反应, 制备了一系列异氰酸酯基修饰的石墨烯, 该功能化石墨烯可以在DMF、NMP、DMSO、HMPA、THF 等非质子溶剂中形成稳定的胶束体系, 并能够长时间保持稳定, 该方法过程简单、条件温和、功能化程度高.除羧基可作为共价修饰的位点外, GO表面的环第6期袁小亚: 石墨烯的制备研究进展 565图5 石墨烯氧化物的共价修饰[85]Fig. 5 Schematic illustration of covalent functionalization of graphene [85]氧基团与羟基也可作为反应的活性点[97-99]. Yang 等[99]利用环氧基团与胺基的亲核取代反应制备表面硅功能化的石墨烯片(图6), 在硅树脂中加入少量该物质能大大改善树脂的力学性能. Satti [93]和Ruoff [100]等利用聚丙烯胺侧链的胺基与GO 表面的环氧基团的反应制备交联的石墨烯, 使得石墨烯薄膜的韧性与强度均有大幅度的提高. 利用高分子化合物主链或侧链的基团与GO 表面或边缘基团的化学反应不仅能改善石墨烯的各种性能, 而且也能制备种类繁多的高性能聚合物−石墨烯纳米复合材 料[101-105]. 石墨烯边缘一些活性双键或缺陷也能发生化学反应如自由基反应[106]、重氮化反应[107-108]、1, 3-偶极加成反应[109], 因此这些部位也能作为石墨烯共价修饰的活性位点.经共价修饰的石墨烯衍生物具有较好的溶解性和可加工性, 但由于杂原子官能团的引入, 破坏了石墨烯的大π共轭结构, 使其导电性与其它性能显著降低, 因此共价修饰的同时如何尽量保持石墨烯的本征性质是一个不容忽视的问题, 为更好地解决此问题, Samulski 与Li 等各自发展了新的共价修饰途径. Samulski 等[110]首先采用硼氢化钠预还原GO, 然后磺化, 最后再用肼还原的方法, 得到了磺酸基功能化的石墨烯. 该方法通过预还原除去了GO 中的多数含氧官能团, 很大程度上恢复了石墨烯的共轭结构, 其导电性显著提高, 而且由于在石墨烯表面引入磺酸基, 使其可溶于水, 便于进一步的研究及应用. Li 等用氨水调节GO 水溶液pH 等于10, 然后用肼还原同样得到导电性高(~7200 S /m)、力学性能好(拉伸模量: 35GPa)、透明性优异(透光率>96%)的石墨烯材料[111], 该法关键之处是控制溶液pH, 在碱性环境(pH=10)中石墨烯表面羧基变成羧酸负离子, 使得石墨烯片与片之间产生较强的静电排斥力(图7), 因此制备的石墨烯水溶液也具有非常好的稳定性.3.3.2 非共价键修饰除了通过在GO 表面上键合一些特定的化学基团来避免还原GO 时石墨烯片层间的重新堆集, 也能利用一些分子与石墨烯之间较强的相互作用力(如π−π堆积力、van der waals 作用力、氢键)来达到稳定单层石墨烯片的效果[85]. 通常这类分子含有较大的芳香环或较强的共轭体系, 能够与大π共轭结构的石墨烯发生较强的相互吸引而被吸附到石墨烯片层上从而得到稳定的胶体分散系统. 芘及其衍生物是一类常用于非共价修饰碳纳米管的共轭结构的分子[112-113], 利用它与石墨烯之间的π−π相互作用, Xu 等研究了芘丁酸对石墨烯的非共价修饰, 使566 无机材料学报第26卷图6 通过环氧位点表面硅功能化的石墨烯片[99]Fig. 6 Silane-functional graphene via chemical reaction on epoxy site[99]图7 化学法制备高分散水溶性石墨烯溶液[111]Fig. 7 Chemical route to the synthesis of aqueous graphene dispersions[111]其在水中形成稳定的分散, 并通过抽滤得到高性能柔性石墨烯薄膜[112]. Stankovich等在还原过程中使用高分子量聚苯乙烯磺酸钠(PSS)对GO表面进行吸附包裹, 避免了团聚, 成功制备了PSS包裹的改性单层石墨烯水溶液[114]. 这是由于PSS 与石墨烯之间有较强的非共价键作用(π−π堆积力), 阻止了石墨烯片的聚集, 使该复合物在水中具有较好的溶解性(1 mg/mL). Hao等[115]用四氰基苯醌作为石墨烯的稳定化剂, 同样获得了能溶于水及有机溶剂(DMSO、DMF) 的非共价修饰的石墨烯. 除利用小分子作为石墨烯的稳定剂外, 一些高分子也能通过非共价作用来修饰石墨烯[73, 116-120]. Li等利用具有大π共轭结构聚苯乙炔类高分子PmPV 与石墨烯之间的相互吸引作用, 制备了PmPV 非共价键修饰的石墨烯带[73].3.4其它方法除上述常用的几种制备石墨烯路线外, 国内外仍不断探索石墨烯新的制备途径. Chakraborty等[121]在成熟的石墨−钾金属复合物基础上制备了聚乙二醇修饰的石墨纳米片, 在有机溶剂及水中均溶解性较好. Wang等[122]利用Fe2+在聚丙烯酸阳离子交换树脂中的配位−掺碳作用, 发展了一种新型的、大规模制备石墨烯的方法: 原位自生模板法(in situ self-generating template), 该法具有产率高、产品晶型好的特点, 制备的石墨烯能作为甲醇燃料电池Pt 催化剂的优良载体. 最近, 复旦大学Feng首先采用Li方法[111]制备石墨烯溶液后, 然后通过高真空(P≈20Pa)低温冷冻干燥制备了高度疏松的粉体石墨烯, 该粉状物只需经简单的超声就能在DMF等有机溶剂中重新形成稳定的胶体分散体系[123], 该法提供了快速简便地大规模制备固态单层石墨烯的途径, 克服了传统方法只能制备分散、稳定石墨烯溶液的缺点, 为石墨烯商业化应用打下了良好基础.4 展望在短短的几年间, 石墨烯以其具有的优异性能及各种潜在的应用前景, 得到快速发掘和开发. 与此同时, 人们需要大量高质量、结构完整的石墨烯材料. 这就要求提高或进一步完善现有制备工艺的水平, 探索新的制备路径. 微机械法显然不能满足未来工业化的要求, 直接剥离法能制备高质量的石墨烯, 但产率太低、耗时太长; 化学气相沉积法可以制备出大面积且性能优异的石墨烯薄膜材料, 但现第6期袁小亚: 石墨烯的制备研究进展 567有的工艺不成熟以及成本较高都限制了其大规模应用, 因此还需进一步探索、完善. 氧化−还原法虽然能够以相对较低的成本制备出大量的石墨烯, 但即使被强还原剂还原后, 石墨烯的原始结构也并不能完全恢复(特别是经过共价修饰后的石墨烯), 而使其电子结构及晶体的完整性均受到严重的破坏,一定程度上限制了其在某些领域(如精密的微电子领域)中的应用. 因此, 如何大量、低成本制备出高质量的石墨烯材料仍是未来研究的一个重点. 此外, 由于表面修饰能改善或丰富石墨烯的各种性能, 也应该关注如何更好的修饰, 特别是非共价修饰,进一步提高石墨烯各方面性能, 促进其器件化、工业化、商品化的进程.参考文献:[1] Novoselov K S, Geim A K, Morozov S V, et al. Electric field effectin atomically thin carbon films. Science, 2004, 306(5696): 666−669.[2] Geim A K, Novoselov K S. The rise of graphene. Nat. Mater., 2007,6(3): 183−191.[3] Geim A K. Graphene: status and prospects. Science,2009,324(5934): 1530−1534.[4] Wu J S, Pisula W, Mullen K. Graphenes as potential material forelectronics. Chem. Rev., 2007, 107(3): 718−747.[5] Rao C N R, Sood A k, Voggu R, et al. Some novel attributes ofgraphene. J. Phys. Chem. Lett., 2010, 1(2): 572−580.[6] Allen M J, Tung V C, Kaner R B. Honeycomb carbon: a review ofGraphene. Chem. Rev., 2010, 110(1): 132−145.[7] Zhang Y, Tan J W, Stormer H L, et al. Experimental observationof the quantum Hall effect and Berry's phase in graphene. Nature, 2005, 438: 201−204.[8] Bolotin K I, Sikes K J, Jiang Z, et al. Ultrahigh electron mobilityin suspended graphene. Solid State Commun., 2008, 146(9/10): 351−355.[9] Balandin A A, Ghosh S, Bao W Z, et al. Superior thermal conduc-tivity of single-layer graphene. Nano Lett., 2008, 8(3): 902−907. [10] Schadler L S, Giannris S C, Ajayan P M. Load transfer in carbonnanotube epoxy composites. Appl. Phys. Lett., 1998, 73(26): 3842−3847.[11] Chae H K, Siberio-Pérez D Y, Kim J, et al. A route to high surfacearea, porosity and inclusion of large molecules in crystals. Nature, 2004, 427: 523−527.[12] Lee C, Wei X, Kysar J W, et al. Measurement of the elastic proper-ties and intrinsic strength of monolayer graphene. Science, 2008, 321(5887): 385−388.[13] Van den Brink J. Graphene-from strength to strength. Nat.Nanotechnol., 2007, 2(4): 199−201.[14] Weitz R T, Yacoby A. Graphene rests easy. Nat. Nanotechnol.,2010, 5(10): 699−700.[15] Kim J, Kim F, Huang J. Seeing graphene-based sheets. Materialstoday, 2010, 13(3): 28−38.[16] Park R, Ruoff R S. Chemical methods for the production of gra-phenes. Nat. Nanotechnol., 2009, 4(4): 217−224.[17] 徐秀娟, 秦金贵, 李振. 石墨烯研究进展. 化学进展, 2009,21(12): 2559−2567.[18] 黄毅, 陈永胜. 石墨烯的功能化及其相关应用. 中国科学B辑,2009, 39(9): 887−896.[19] 李旭, 赵卫峰, 陈国华. 石墨烯的制备与表征研究. 材料导报,2008, 22(8): 48-52.[20] Müllen M, Kübel C, Müllen K. Giant polycyclic aromatic hydro-carbons. Chem. Eur. J., 1998, 4(11): 2099−2109.[21] Stankovich S, Dikin D A, Piner R D, et al. Synthesis of graphene-based nanosheets via chemical reduction of exfoliated graphite ox-ide. Carbon, 2007, 45(7): 1558−1565.[22] Hernandez Y, Nicolosi V, Lotya M, et al. High-yield production ofgraphene by liquid-phase exfoliation of graphite. Nat. Nanotech-nol., 2008, 3(9): 563−568.[23] Khan U, O'Neill A, Lotya M, et al. High-concentration solvent ex-foliation of graphene. Small, 2010, 6(7): 864−871.[24] Hamilton C E, Lomeda J R, Sun Z, et al. High-yield organic dis-persions of unfunctionalized graphene. Nano Lett., 2009, 9(10): 3460−3462.[25] Biswas S, Drzal L T. A novel approach to create a highly orderedmonolayer film of graphene nanosheets at the liquid−liquid inter-face. Nano Lett., 2009, 9(1): 167−172.[26] Qian W, Hao R, Hou Y, et al. Solvothermal-assisted exfoliationprocess to produce graphene with high yield and high quality.Nano Res., 2009, 2: 706−712.[27] Lotya M, Hernandez Y, King P J, et al. Liquid phase production ofgraphene by exfoliation of graphite in surfactant/water solutions.J.Am. Chem. Soc., 2009, 131(10): 3611−3620.[28] De S, King P J, Lotya M, et al. Flexible, transparent, conductingfilms of randomly stacked graphene from surfactant-stabilized, oxide-free graphene dispersions. Small, 2010, 6(3): 458−464. [29] Englert J M, Röhrl J, Schmidt C D, et al. Soluble graphene: genera-tion of aqueous graphene solutions aided by a perylenebisimide- based bolaamphiphile.Adv. Mater., 2009, 21(42): 4265−4269. [30] Li X, Zhang G, Bai X, et al. Highly conducting graphene sheetsand Langmuir–Blodgett films. Nat. Nanotechnol., 2008, 3(9): 538−542.[31] Janowska I, Chizari K, Ersen O, et al. Microwave synthesis oflarge few-layer graphene sheets in aqueous solution of ammonia.Nano Res., 2010, 3(2): 126−137.[32] Pu N W, Wang C, Sung Y, et al. Production of few-layer grapheneby supercritical CO2 exfoliation of graphite. Mater. Lett., 2009, 63(23): 1987−1989.[33] Knieke C, Berger A, Voigt M, et al. Scalable production of gra-phene sheets by mechanical delamination. Carbon, 2010, 48(11):。
石墨烯表面活性剂

序号分散剂/活性剂的名称简介结构1N-甲基-吡咯化合物(烷酮)2十二烷基苯磺酸钠(SDBS)分子式:C18H29NaO3S;分子量:348.48;固体,白色或淡黄色粉末,易溶于水,易吸潮结块;无毒3聚乙烯吡咯烷酮(PVP)分子式:(C6H9NO)n;白色或乳白色粉末或颗粒;4阴离子型表面活性剂:木质素磺酸钠(SLS)多聚物;分子量不定;化学结构尚未确定5胆酸钠(SC)分子式:C24H39Nao5;分子量:430.55;白色结晶或无色粉末6高浓度的胆酸钠7十六烷基三甲基溴化铵(CTAB)分子式: C16H33(CH3)3NBr;分子量:364.446;白色微晶性粉末,吸湿性,在酸性溶液中稳定,易溶于乙醇8聚氧乙烯月桂醚(Brij 35)棕色粘稠液,易溶于水,具有乳化、润湿、分散能力标准情况下熔点约为27℃沸点约100℃密度接近于水约为1.00g/mL,闪点大于110℃折射率1.462可以用作乳化润湿剂,在橡胶工业中用作分散剂,石油工业和环境保护行业中用作溢油分散剂的组分之一。
也可用作醚酯类非离子型表面活性剂9吐温80分子式:C64H124O26 ;分子量:1309.5 ;浅黄色粘稠液体10曲拉通X100聚乙二醇对异辛基苯基醚;分子式:C34H62O11;分子量:646.86;对人体有害。
11氧化二丁基锡分子式:C8H18OSn ,分子量:248.95 ,白色到微黄色粉末。
熔点 >300℃,水溶性 4.0 mg/L(20℃)。
溶于盐酸,不溶于水及有机溶剂。
遇火自燃;剧毒12Disperbyk-163分散剂 Disperbyk-163 是一种相对分子质量为 17000,的嵌段共聚物,包括亲颜料端的胺基和亲溶剂端的酯基和羧基。
13赖氨酸14聚间亚苯亚乙烯衍生物(PmPV)15DsPE16聚乙烯醇(PVA) 17聚丙烯酰胺剂制备方法;CN101941694。
石墨烯_氧化钌纳米复合材料的水热法合成及电化学电容性能_英文_沈辰飞

收稿日期:2010-08-03。
收修改稿日期:2010-11-10。
江苏省自然科学基金(No.BK2006195)资助项目。
*通讯联系人。
E -mail :jmcao@石墨烯-氧化钌纳米复合材料的水热法合成及电化学电容性能沈辰飞1郑明波2薛露平1李念武1吕洪岭1张松涛1曹洁明*,1(1南京航空航天大学材料科学与技术学院纳米材料研究所,南京210016)(2南京大学微结构国家实验室电子科学与工程学院,南京210093)摘要:通过水热法制备了石墨烯-氧化钌(G -RuO 2)纳米复合材料。
对样品进行了X 射线衍射(XRD),扫描电子显微镜(SEM),透射电子显微镜(TEM)和能量色散谱(EDS)表征。
SEM 结果表明氧化钌粒子均匀地分散在石墨烯层片上。
TEM 结果显示氧化钌纳米粒子的平均粒径约为3nm 。
对样品进行了循环伏安和充放电性能测试,结果表明在1A ·g -1的电流密度下,样品在H 2SO 4(1mol ·L -1)溶液中具有219.7F ·g -1的比电容。
关键词:氧化钌;石墨烯;纳米复合材料;电化学电容器;水热中图分类号:O613.71;O614.82+1文献标识码:A文章编号:1001-4861(2011)03-0585-05Graphene -RuO 2Nanocomposites:Hydrothermal Synthesis and ElectrochemicalCapacitance PropertiesSHEN Chen -Fei 1ZHENG Ming -Bo 2XUE Lu -Ping 1LI Nian -Wu 1L 譈Hong -Ling 1ZHANG Song -Tao 1CAO Jie -Ming *,1(1Nanomaterials Research Institute,College of Materials Science and Technology,Nanjing University ofAeronautics and Astronautics,Nanjing 210016,China )(2National Laboratory of Microstructures,School of Electronic Science and Engineering,Nanjing University,Nanjing 210093,China )Abstract:Graphene -ruthenium oxide (G -RuO 2)nanocomposite was prepared via a facile hydrothermal method.The sample was characterized by X -ray diffraction (XRD),scanning electron microscopy (SEM),transmission electron microscopy (TEM)and energy dispersive X -ray Spectroscopy (EDS).SEM result reveals homogeneous distribution of RuO 2particles on the layers of graphene sheets.TEM images demonstrate that the average size of RuO 2particles is around 3nm.The electrochemical properties of the sample were examined by cyclic voltammetry (CV)and galvanostatic charge -discharge (GC).The specific capacitance value of the sample is about219.7F·g -1at the current density of 1A ·g -1in 1mol ·L -1H 2SO 4.Key words:ruthenium oxide;graphene;nanocomposites;electrochemical capacitor;hydrothermal method0IntroductionElectrochemical capacitors (ESCs)have attracted attention as electricity storage devices due to their higher power capability and longer cycle life compared with conventional double -layer capacitors [1-2].With longcyclelife and high specic capacitance,metal oxide and carbonaceous materials have been viewed as promising electrode materials for ESCs.Metal oxides such as RuO 2[3-7],MnO 2[8-9],NiO [10-11]and Co 3O 4[12-13]have been investigated for their electrochemical behaviors.Among them,electrochemical capacitors based on RuO 2第27卷第3期2011年3月Vol .27No .3585-589无机化学学报CHINESE JOURNAL OF INORGANIC CHEMISTRY第27卷无机化学学报electrode have been widely investigated due to their superior specfic capacitance,high electrochemical reversibility,and long cycle life[14-16].In terms of carbonaceous materials,carbon nanotubes[17-18],carbon fibers[19],carbon aerogels[20]and activated carbons[21]have been studied for their electrochemical behaviors.Graphene,a new kind of carbonaceous material,has been reported with unique properties and thus has drew great interests[22-23]. Vivekchand et al.[24]evaluated the capacitive behaviors of graphene and a capacitive performance of graphene up to117F·g-1in aqueous H2SO4solution was obtained.Stoller et al.[25]studied the electrochemical behaviors of graphene as the electrode of ESCs and a specfic capacitance of135F·g-1in5.5mol·L-1KOH aqueous electrolyte was achieved.Du et al.[26]used graphene as the electrode of ESCs to obtain a stable specfic capacitance of150F·g-1under specific current of0.1A·g-1for500cycles of charge/discharge. Recently,Du et al.[27]prepared functionalized graphene sheets(FGS)using graphite oxide(GO)as precursor via a low-temperature thermal exfoliation approach in air and the products exhibited good electrochemical behaviors.To harness the good electrochemical properties of both metal oxide and graphene sheets,one possible route is to integrate these two kinds of materials into the electrodes of ESCs.The capacitive performance of the composites will be enhanced largely because most of the metal oxide can contribute pseudo-capacitance to the total capacitance apart from the double-layer capacitance from graphene sheets[28-29].Yan et al.[30] synthesized graphene-MnO2composites through the self-limiting deposition of nanoscale MnO2on the surface of graphene under microwave irradiation and studied the electrochemical behaviors of the products. Son et al.[31]fabricated NiO Resistive Random Access Memory(RRAM)nanocapacitor array on a graphene sheet.In this work,we synthesized graphene-RuO2 nanocomposite with15wt%RuO2loading via a facile hydrothermal method and studied electrochemical characteristics of the products in an aqueous electrolyte.1Experimental1.1Preparation of FGSGO was prepared by Hummers method[32].To obtain FGS,certain amount of GO was thermally exfoliated at300℃for5min under air atmosphere and denoted as FGS300[27].1.2Preparation of G-RuO2All reagents were analytical grade and used without further purification.To prepare the sample with 15wt%RuO2loading of the composite,60mg FGS300 was dissolved in60mL distilled water.After vigorous stirring,a stable suspension was obtained.1.7mL of RuCl3(0.048mol·L-1)was then dropped into the suspension with ultrasonication for30min.Then,the solution was transferred into a Teflon-lined autoclave with a capacity of100mL,and then the autoclave was sealed and maintained at180℃for6h.After cooling to room temperature,the black product was washed with distilled water for several times,and dried under vacuum at50℃for48h.1.3CharacterizationThe dimension and the morphology of the sample were observed by SEM(Gemini LEO1530)and TEM (JEOL JEM-2100).Composition of the samples was analyzed using TEM attached energy dispersive X-ray spectroscopy(EDS).XRD patterns were recorded by a Bruker D8-Advance diffractometer using Cu Kαradiation(λ=0.15418nm)with the scanning2θangles ranging from10°to80°,a graphitic monochrom at40 kV and40mA.1.4Electrochemical testsCyclic voltammetry(CV)and galvanostatic charge-discharge(GC)were done in a three-electrode experimental setup using1mol·L-1H2SO4as electrolyte.The prepared electrode,platinum foil,and saturated calomel electrode(SCE)were used as the working,counter,and reference electrodes in1mol·L-1 H2SO4aqueous solution.The preparation of the working electrode for the three-electrode system was as follows: 80wt%G-RuO2sample,15wt%acetylene black,and 5wt%polytetrafluoroethylene were well mixed,then the mixture was pressed onto a stainless steel grid under10586第3期沈辰飞等:石墨烯-氧化钌纳米复合材料的水热法合成及电化学电容性能MPa.Each electrode contained 5.0mg of G-RuO2(active material).The CV and GC measurements werecarried out on CHI440A electrochemical workstation atroom temperature,the potential ranging from0V to1.0V(vs.SCE).The GC measurement was carried out incurrent density range of0.5~5A·g-1.2Results and discussionFig.1shows the XRD patterns of FGS300and G-RuO2nanocomposite.The characteristic(002)peak ofFGS300is clearly observed on the XRD patterns ofboth FGS300and G-RuO2[27].From a comparison ofthese two XRD patterns,no obvious diffraction peakscorresponding to RuO2are found in G-RuO2.It is supposed to be due to the small size of the RuO2 particles.Fig.2(a)shows the SEM image of FGS300.The wrinkle,a characteristic feature of graphene sheets,is observed.RuO2particles are observed decorated on the graphene sheets from Fig.2(b)and Fig.2(c).The uniform distribution of RuO2particles on graphene sheets guarantees the good electrochemical properties of G-RuO2[33].The HRTEM image(Fig.3(d))reveals the good crystalline nature of the nanoparticles.Besides, the size of the RuO2particles is observed to be around 3nm,which explains the low intensity of diffraction peaks of RuO2in the XRD pattern of the composite. The selected area electron diffraction(SAED)pattern in Fig.2(e)shows a ring pattern,indicating that the obtained RuO2particles are polycrystalline,which is consistent with the HRTEM observation.Moreover,the first ring matches the(110)plane of RuO2.The other rings are very close to both structures of Ru and RuO2,which may be due to incomplete oxidation of RuCl3[34].The EDS spectrum(Fig.1(f))reveals the existence of Ru and O species,of which the Ru and O elements should be the main contribution of RuO2 phase in the composite.CV and GC were used to investigate electrochemical behaviors of G-RuO2in a three-electrode system in1mol·L-1H2SO4electrolyte.Fig.3 (a)shows the CV curves of G-RuO2at different scan rates(5~50mV·s-1)in the potential range from0to1.0 Fig.1XRD patterns of FGS300and G-RuO2nanocompositeFig.2SEM images of FGS300and G-RuO2nanocomposite(a,b),TEM and HRTEM images of G-RuO2nanocomposite(c,d), SAED pattern of G-RuO2composite(e),EDS spectrum of G-RuO2composite(f)587第27卷无机化学学报Table 1Specific capacitances of G -RuO 2(C s,composite )obtained in 1mol ·L -1H 2SO 4from GC method andcapacitance retention for G -RuO 2V.Broad current peaks and almost mirror quasi -rectangular are observed in all the CV curves over theCV potential range.This indicates that the obtained G -RuO 2nanocomposite exhibits high redox reversibility and obvious pseudocapacitance character.[35]Galvanostatic cycling of G -RuO 2is performed ata current density of 0.5~5A ·g -1as shown in Fig.3(b).The specific capacitance of G -RuO 2(C s,composite )could be calculated from the slope of the charge -dischargecurves,according to the equation:C s,composite =I Δt [36],where I is the current of charge -discharge,Δt is the time of discharge,m is the mass of active materials in the working electrode,and ΔV is 1.0V.The calculated specfic capacitances of G -RuO 2at different scan rates and the capacitance retention of the samples are listed in Table 1.The results demonstrate high capacitance retention of the sample.Besides,atthe current density of 1A ·g -1,G -RuO 2exhibits capacitance value of 219.7F ·g -1,which is muchhigher than that of FGS300(119.1F ·g -1).The specific capacitance of RuO 2(C s,Ru )could be calculated basedon the equation:C s,Ru =C s,composite -(1-ωRu )C s,FGS300ωRu[33],where ωRu is the weight fraction of RuO 2within the nanocomposite,C s,FGS300is the specific capacitance of FGS300.At the current density of 1A ·g -1,C s,Ru iscalculated to be 789.8F·g -1.The distinguishing electrochemical behaviors of G -RuO 2are due to the excellent electrochemical properties of FGS300and the contribution of pseudocapacitance by RuO 2.With nanoporous structure,the obtained FGS300has fully accessible surface to electrolyte ion because both sides of a broad range of graphene sheets can be exposed to the electrolyte and contribute to capacitance [27].Furthermore,the residual functional groups on the surface of FGS may improve the hydrophilicity of electrode,which helps the RuO 2particles to be loaded onto the surface of FGS300.The RuO 2and the residual functional groups on graphene sheets all contribute to the pseudocapacitance and thus enhance the overall capacitance value of the composites.Fig.3(a)Cyclic voltammograms of G -RuO 2obtained at different scan rates.(b)Galvanostatic dischargecurves of G -RuO 2obtained at different current densities and FGS300at a current density of 1A ·g -1G -RuO 2233.6219.7200.7176.875.70.5A·g -11A ·g -12A ·g -15A ·g -1Sample C s,composite /(F ·g -1)Capacitance retention /%3ConclusionIn summary,graphene -RuO 2nanocomposite wasprepared via a facile hydrothermal method.The SEMand TEM characterizations reveal homogeneous distribution of RuO 2particles on graphene sheets.High capacitance value and capacitance retention of the composite are shown by capacitive behaviors of G -588第3期RuO2.References:[1]Winter M,Brodd R J.Chem.Rev.,2004,104:4245-4269[2]Pandolfo A G,Hollenkamp A F.J.Power Sources,2006,157:11-27[3]WANG Xiao-Feng(王晓峰),WANG Da-Zhi(王大志),LIANGJi(梁吉),et al.Acta Phys.-Chim.Sin.(Wuli Huaxue Xuebao), 2002,18(8):750-753[4]Hu C C,Chen W C,Chang K H.J.Electrochem.Soc.,2004,151:A281-A290[5]Fang W C,Huang J H,Chen L C,et al.J.Power Sources,2006,160:1506-1510[6]Sugimoto W,Yokoshima K,Murakami Y,et al.Electrochim.Acta,2006,52:1742-1748[7]ZHENG Yan-Zhen(郑言贞),ZHANG Mi-Lin(张密林),CHEN Ye(陈野),et al.Chinese J.Inorg.Chem.(Wuji Huaxue Xuebao),2007,23(4):630-634[8]Toupin M,Brousse T,Belanger D.Chem.Mater.,2004,16:3184-3190[9]Lee H Y,Goodenough J B.J.Solid State Chem.,1999,144:220-223[10]Zheng Y Z,Ding H Y,Zhang M L.Mater.Res.Bull.,2009,44:403-407[11]Patil U M,Salunkhe R R,Gurav K V,et al.Appl.Surf.Sci.,2008,255:2603-2607[12]Cui L,Li J,Zhang X G.J.Appl.Electrochem.,2009,39:1871-1876[13]Shinde V R,Mahadik S B,Gujar T P.Appl.Surf.Sci.,2006,252:7487-7492[14]Zheng J P,Jow T R.J.Electrochem.Soc.,1995,142:L6-L8[15]Zheng J P,Jow T R.J.Power Sources,1996,62:155-159[16]Sugimoto W,Iwata H,Murakami Y,et al.J.Electrochem.Soc.,2004,151:A1181-A1187[17]Ma R Z,Liang J,Wei B Q,et al.Bull.Chem.Soc.Jpn.,1999,72:2563-2566[18]MI Hong-Yu(米红宇),ZHANG Xiao-Gang(张校刚),L譈Xin-Mei(吕新美),et al.Chinese J.Inorg.Chem.(Wuji Huaxue Xuebao),2007,23(1):159-163[19]Xu B,Wu F,Chen R J,et al.J.Power Sources,2010,195:2118-2124[20]Li J,Wang X Y,Huang Q H,et al.J.Power Sources,2006,158:784-788[21]Bispo-Fonseca I,Aggar J,Sarrazin C,et al.J.Power Sources,1999,79:238-241[22]Novoselov K S,Geim A K,Morozov S V,et al.Science,2004,306:666-669[23]Stankovich S,Dikin D A,Dommett G H B,et al.Nature,2006,442:282-286[24]Vivekchand S R C,Rout C S,Subrahmanyam K S,et al.J.Chem.Sci.,2008,120:9-13[25]Stoller M D,Park S J,Zhu Y W,et al.Nano Lett.,2008,8:3498-3502[26]Du X,Guo P,Song H H,et al.Electrochim.Acta,2010,55:4812-4819[27]Du Q L,Zheng M B,Zhang L F,et al.Electrochim.Acta,2010,55:3897-3903[28]Kalpana D,Omkumar K S,Kumar S S,et al.Electrochim.Acta,2006,52:1309-1315[29]Lee B J,Sivakkumar S R,Ko J M,et al.J.Power Sources,2007,168:546-552[30]Yan J,Fan Z J,Wei T,et al.Carbon,2010,48:3825-3833[31]Son J Y,Shin Y H,Kim H,et al.ACS Nano,2010,4:2655-2658[32]Hummers W S,Offeman R E.J.Am.Chem.Soc.,1958,80:1339-1339[33]Yuan C Z,Chen L,Gao B,et al.J.Mater.Chem.,2009,19:246-252[34]Fang W C,Chyan O,Sun C L,et mun.,2007,9:239-244[35]Wen J G,Zhou Z T.Mater.Chem.Phys.,2006,98:442-446[36]Zheng M B,Cao J,Liao S T,et al.J.Phys.Chem.C,2009,113:3887-3894沈辰飞等:石墨烯-氧化钌纳米复合材料的水热法合成及电化学电容性能589。
氧化石墨烯的制备及其在防腐蚀导热涂料中的应用
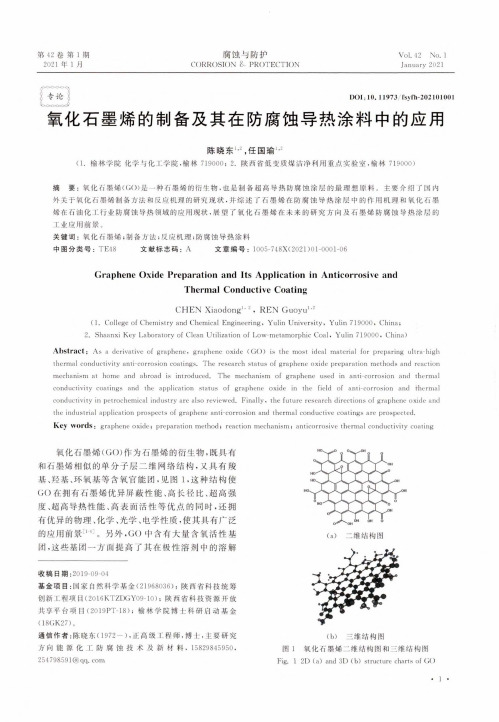
第42卷第1期2021年1月Vol.42No.1January2021腐蚀与防护CORROSION&PROTECTION^>oo<xxPDOI:10.11973fsyfh-202101001氧化石墨烯的制备及其在防腐蚀导热涂料中的应用陈晓东',任国瑜t(1.榆林学院化学与化工学院,榆林719000; 2.陕西省低变质煤洁净利用重点实验室,榆林719000)摘要:氧化石墨烯(GO)是一种石墨烯的衍生物,也是制备超高导热防腐蚀涂层的最理想原料。
主要介绍了国内外关于氧化石墨烯制备方法和反应机理的研究现状•并综述了石墨烯在防腐蚀导热涂层中的作用机理和氧化石墨烯在石油化工行业防腐蚀导热领域的应用现状•展望了氧化石墨烯在未来的研究方向及石墨烯防腐蚀导热涂层的工业应用前景。
关键词:氧化石墨烯;制备方法;反应机理;防腐蚀导热涂料中图分类号:TE48文献标志码:A文章编号:1005-748X(2021)01-0001-06Graphene Oxide Preparation and Its Application in Anticorrosive andThermal Conductive CoatingCHEN Xiaodong1-2,REN Guoyu1*2(1.College of Chemistry and Chemical Engineering.Yulin University,Yulin719000»China;2.Shaanxi Key Laboratory of Clean Utilization of Low-metamorphic Coal»Yulin719000,China)Abstract:As a derivative of graphene,graphene oxide(GO)is the most ideal material for preparing ultra-high thermal conductivity anti-corrosion coatings.The research status of graphene oxide preparation methods and reaction mechanism at home and abroad is introduced.The mechanism of graphene used in anti-corrosion and thermal conductivity coatings and the application status of graphenc oxide in the field of anti-corrosion and thermal conductivity in petrochemical industry are also reviewed.Finally*the future research directions of graphene oxide and the industrial application prospects of graphene anti-corrosion and thermal conductive coatings are prospected.Key words:graphene oxide;preparation method;reaction mechanism;anticorrosive thermal conductivity coating氧化石墨烯(GO)作为石墨烯的衍生物,既具有和石墨烯相似的单分子层二维网络结构,又具有竣基、径基、环氧基等含氧官能团,见图1,这种结构使G()在拥有石墨烯优异屏蔽性能、高长径比、超高强度、超高导热性能、高表面活性等优点的同时.还拥有优异的物理、化学、光学、电学性质,使其具有广泛的应用前景另外,G()中含有大量含氧活性基团,这些基团一方面提高了其在极性溶剂中的溶解收稿日期:2019-09-04基金项目:国家自然科学基金(21968036);陕西省科技统筹创新工程项目(2O16KTZDGYO9-1O);陕西省科技资源开放共享平台项目(2019PT-18);榆林学院博士科研启动基金(18GK27).通信作者:陈晓东(1972—),正高级工程师,博士,主要研究方向能源化工防腐蚀技术及新材料.158****5950, 254798591@(b)三维结构图图1氧化石墨烯二维结构图和三维结构图Fig.12D(a)and3D(b)structure charts of GO•1•陈晓东.等:氧化石墨烯的制备及其在防腐蚀导热涂料中的应用性,另一方面也有利于对石墨烯进行共价键功能化,使其成为进一步功能化的最佳原料和制备超高导热防腐蚀涂层最理想的原料“切。
Graphene-based composite materials

Graphene-based composite materialsSasha Stankovich 1*,Dmitriy A.Dikin 1*,Geoffrey H.B.Dommett 1,Kevin M.Kohlhaas 1,Eric J.Zimney 1,Eric A.Stach 3,Richard D.Piner 1,SonBinh T.Nguyen 2&Rodney S.Ruoff 1Graphene sheets —one-atom-thick two-dimensional layers of sp 2-bonded carbon —are predicted to have a range of unusual properties.Their thermal conductivity and mechanical stiffness may rival the remarkable in-plane values for graphite (,3,000Wm 21K 21and 1,060GPa,respectively);their fracture strength should be comparable to that of carbon nanotubes for similar types of defects 1–3;and recent studies have shown that individual graphene sheets have extraordinary electronic trans-port properties 4–8.One possible route to harnessing these proper-ties for applications would be to incorporate graphene sheets in a composite material.The manufacturing of such composites requires not only that graphene sheets be produced on a sufficient scale but that they also be incorporated,and homogeneously distributed,into various matrices.Graphite,inexpensive and available in large quantity,unfortunately does not readily exfoliate to yield individual graphene sheets.Here we present a general approach for the preparation of graphene-polymer composites via complete exfoliation of graphite 9and molecular-level dispersion of individual,chemically modified graphene sheets within poly-mer hosts.A polystyrene–graphene composite formed by this route exhibits a percolation threshold 10of ,0.1volume per cent for room-temperature electrical conductivity,the lowest reported value for any carbon-based composite except for those involving carbon nanotubes 11;at only 1volume per cent,this composite has a conductivity of ,0.1S m 21,sufficient for many electrical applica-tions 12.Our bottom-up chemical approach of tuning the graphene sheet properties provides a path to a broad new class of graphene-based materials and their use in a variety of applications.Graphite oxide is a layered material produced by the oxidation of graphite (Fig.1a).In contrast to pristine graphite,the graphene-derived sheets in graphite oxide (graphene oxide sheets)are heavily oxygenated,bearing hydroxyl and epoxide functional groups on their basal planes,in addition to carbonyl and carboxyl groups located at the sheet edges 13,14.The presence of these functional groups makes graphene oxide sheets strongly hydrophilic,which allows graphite oxide to readily swell and disperse in water 2,15,16.Previous studies by our team have shown that a mild ultrasonic treatment of graphite oxide in water results in its exfoliation to form stable aqueous dispersions that consist almost entirely of 1-nm-thick sheets (Fig.1b)9.Given the uniformity of the observed thicknesses and that platelets one-half of the observed minimum thickness are never detected (or any other inverse integer value,such as one-third,and so on),we believe that these represent fully exfoliated graphene oxide sheets.In fact,at present,exfoliation of graphite oxide is the only way to produce stable suspensions of quasi-two-dimensional carbon sheets,making this a strategic starting point for large-scale synthesis of graphene sheets.As such,graphite oxide has recently attracted attention as a filler for polymer nanocomposites 17–19.Nanocomposites have been heralded 20as a ‘radical alternative toconventional filled polymers or polymer blends,’especially in the fields of transportation and electronics.Unfortunately,owing to their hydrophilic nature,graphene oxide sheets can only be dispersed in aqueous media that are incompatible with most organic polymers.In addition,graphite oxide is electrically insulating,unlike graphite,which limits its usefulness for the synthesis of conductive nanocom-posites.It has been demonstrated 9,18,21,22,however,that the electrical conductivity of graphite oxide can be significantly increased by chemical reduction,presumably owing to the restoration of a graphitic network of sp 2bonds.But reduction of exfoliated graphene oxide nanoplatelets in water results in their irreversible coagulation 9,which then makes dispersion within a polymer matrix at the individual sheet level impossible.We recently demonstrated that the exfoliation behaviour of graphite oxide can be altered by changing the surface properties of graphene oxide sheets by way of chemical functionalization.A number of chemically modified graphite oxides were prepared by treating graphite oxide with organic isocyanates 23.The isocyanate treatment reduces the hydrophilic character of graphene oxide sheets by forming amide and carbamate ester bonds to the carboxyl and hydroxyl groups of graphite oxide,respectively.As a result,such isocyanate-derivatized graphite oxides no longer exfoliate in water but readily form stable dispersions in polar aprotic solvents (such as N ,N -dimethylformamide (DMF)),consisting of completely exfoliated,functionalized individual graphene oxide sheets with thickness ,1nm,as determined by atomic force microscopy,AFM (Fig.1c).These dispersions of isocyanate-derivatized graphite oxide allow graphene oxide sheets to be intimately mixed with many organic polymers,facilitating synthesis of graphene–polymer composites.Coupled with the possibility that graphite oxide can be chemically reduced (see above),we were hopeful that nanocomposites of polymer and isocyanate-derivatized graphite oxide could be rendered electrically conductive.Hence,we were gratified to find that electrically conductive graphene–polymer nanocomposites can be prepared by solution-phase mixing of the exfoliated phenyl isocyanate-treated graphite oxide sheets with polystyrene,followed by their chemical reduction (Fig.1d).These composites feature individual graphene sheets well dispersed throughout the polymer matrix (Fig.1g).Similar disper-sions have been achieved with other styrenic polymers such as acrylonitrile-butadiene-styrene and styrene-butadiene rubbers.Chemical reduction was essential for inducing electrical conduc-tivity,as composite samples with un-reduced phenyl isocyanate-treated graphite oxide sheets were insulating.In addition,the presence of the polymer in solution during the reduction step was key to preventing the agglomeration of the sheets.As the reduction proceeds,the sheets become coated with the polymer and remain individually dispersed (Fig.1d).When the reduction step preceded the introduction of the polymer,significant agglomeration of grapheneLETTERS1Department of Mechanical Engineering,2Department of Chemistry,Northwestern University,2145Sheridan Road,Evanston,Illinois 60208-3111,USA.3School of Materials Engineering and Birck Nanotechnology Center,Purdue University,501Northwestern Avenue,West Lafayette,Indiana 47907,USA.*These authors contributed equally to thiswork.sheets occurred.Further details of the preparation and fabrication of composite samples are described in Methods.In a concurrent research direction,we explored a rapid thermal expansion of graphite oxide(,1,0008C under inert gas; R.K.Prud’homme et al.,manuscript in preparation).However,the thermally expanded graphite oxide platelets so produced are not fully exfoliated,consisting of several-nanometre-thick multilayer stacks, and their dispersion in a polymer matrix is a challenging step of manufacture.For the purpose of comparison,polystyrene compo-sites prepared with such thermally expanded graphite oxide,by the same procedure as was used for phenyl isocyanate-treated graphite oxide,are less homogeneous and have higher electrical percolation thresholds(see Supplementary Information).For this and other reasons,we have focused on the bottom-up approach that yields molecular-level dispersion of individual graphene sheets as observed by scanning electron microscopy,SEM(Fig.1g).The quality of nanofiller dispersion in the polymer matrix directly correlates with its effectiveness for improving mechanical,electrical, thermal,impermeability and other properties.The properties of a composite are also intimately linked to the aspect ratio and surface-to-volume ratio of thefiller.The potential properties of our graphene-sheet-based composites thus appear promising owing to the extremely high aspect ratios of the sheets as determined from SEM images (Fig.1g),in which the average lateral dimension was estimated to be,1m m,similar to the values found by AFM(Fig.1b,c).However, in contrast to the typicallyflat sheets seen by AFM when deposited onto atomicallyflat substrates,the sheets in the composites are crumpled and wrinkled,and at times folded.At2.4volume per cent(vol.%)loading,the composite appears in the SEM images to be almost entirelyfilled with the graphene sheets, even though97.6vol.%is stillfilled by the polymer(Fig.1g,right panel).This‘visual’effect is due to the enormous surface area of the sheets.The specific surface area of an individual graphene sheet is ,2,600m2g21,so1m m3of a composite with1vol.%graphene sheets would have,50m m2of sheet surface area.SEM image analysis was used to estimate the apparent graphene sheet surface area at different concentrations(Fig.2a–d).It revealed a surface area lower by roughly a factor of two than the calculated values for each concentration.The nanoscale corrugation of the graphene sheets(not evident in SEM imaging)may contribute to the discrepancy.The intrinsic mechanics of the sheets,their crumpling,wrinkling and folding,and whether they can be processed to be non-crumpled(for example,sothatFigure1|Processflow of graphene–polymer composite fabrication.a,SEM and digital image(inset)of natural graphite.b,A typical AFM non-contact-mode image of graphite oxide sheets deposited onto a mica substrate from an aqueous dispersion(inset)with superimposed cross-section measurements taken along the red line indicating a sheet thickness of ,1nm.c,AFM image of phenyl isocyanate-treated graphite oxide sheets on mica and profile plot showing the,1nm thickness.d,Suspension of phenyl isocyanate-treated graphite oxide(1mg ml21)and dissolved polystyrene in DMF before(left)and after(right)reduction by N,N-dimethylhydrazine. e,Composite powder as obtained after coagulation in methanol.f,Hot-pressed composite(0.12vol.%of graphene)and pure polystyrene of the same0.4-mm thickness and processed in the same way.g,Low(top row) and high(bottom row)magnification SEM images obtained from a fracture surface of composite samples of0.48vol.%(left)and2.4vol.%(right) graphene in polystyrene.NATURE|Vol442|20July2006LETTERSlayering could be more effectively achieved)are issues for further study.Heat treatment of such composites to 3008C,well above the glass transition temperature of polystyrene (1008C),does not result in agglomeration of the graphene sheets (as assessed from extensive SEM imaging),suggesting their thermal stability over a broad temperature range.As SEM cannot spatially resolve the thickness of an individual graphene-based sheet,transmission electron microscopy (TEM)was used to determine if the graphene-based sheets were indeed present in the composites as single,exfoliated sheets or as multi-layered platelets.Extensive high-resolution phase-contrast imaging showed no evidence of multi-layer stacks,even though SEM of the same slices clearly indicated the presence of nanoplatelets.In contrast,selected-area electron diffraction (SAED)of composite samples yielded spot patterns matching those expected for individual graphene-based sheets (Fig.2e,f,and Supplementary Information).SAED of pure polystyrene samples fabricated in an identical manner (see Methods)showed the ring pattern expected for an amorphous material.The conductive nature of the graphene filler motivated our study of the percolation behaviour through electrical measurements.As a control,we prepared polystyrene composites containing phenyl isocyanate-treated graphite oxide sheets that were not chemically reduced.At similar graphene concentrations,these samples were greyish in colour compared to the almost black composites filled with the reduced material,and they were not electrically conductive.To obtain the true value of the conductivity of the composites,we made separate measurements of in-plane and transverse resistances(Fig.3,inset)and developed a numerical simulation program based on FEMLAB (version 3.1,Comsol AB),which takes into account geometry of the samples and electrical leads.All measurements and subsequent analysis revealed minor anisotropy,with the in-plane conductivity,j k ,always slightly higher than the transverse conductivity,j ’.A rapid increase in the direct current electrical conductivity of composite materials takes place when the conductive filler forms an infinite network of connected paths through the insulating matrix.When the filler particles are rigid bodies,the conductivity of such media is typically described with a bond percolation model 10.The conductivity of the composite,j c ,above the percolation threshold is then treated with a power law:j c ¼j f [(f 2f c )/(12f c )]t (ref.24),where j f is the conductivity of the filler,f the filler volume fraction,f c the percolation threshold (the onset of the transition),and t is the ‘universal critical exponent’.Percolation in polystyrene–graphene composites occurs when the filler concentration,f c ,is near 0.1vol.%(Fig.3).This percolation threshold is,to the best of our knowledge,about three times lower than reported for any other two-dimensional filler 25.Such a low percolation threshold for a three-dimensional isotropic case is evidently due to the extremely high aspect ratio of the graphene sheets and their excellent homogeneous dispersion in these compo-sites.Randomly oriented oblate ellipsoids (disks)with an aspect ratio of 1,000are predicted to have a geometric percolation threshold of ,0.1vol.%(ref.26).At a loading of about 0.15vol.%,the conduc-tivity of our composites already satisfies the antistatic criterion (1026S m 21)for thin films 12and rapidly rises over a 0.4vol.%range.An increase in graphene sheet loading above 0.5vol.%yields a more gradual increase in electrical conductivity,with values of ,0.1S m 21at 1vol.%and ,1S m 21at 2.5vol.%.At present,research in nanocomposite materials using carbon-based nanofillers is dominated by carbon nanotubes.The electrical properties of our composites compare well with the best values reported in the literature for nanotube–polymer composites.TheFigure 2|SEM and TEM images of graphene–polystyrene composite.a –d ,SEM images of the microtomed composites reveal differentmorphologies of the graphene sheets,including their packing,at different concentrations (vol.%):a ,0.24;b ,0.96;c ,1.44;and d ,2.4.Scale bar,shown in a ,applies to a –d .e ,f ,High-resolution phase contrast images and SAED patterns (inset)of e ,cast film made from powder composite,and f ,microtomed composite sample.The SAED patterns show the six-foldrotational symmetry expected for diffraction with the beam incident along [0001].Our experimentally obtained patterns show all of the reflections expected for such single graphene-based sheets.The d spacings associated with the spots were determined using both gold and graphite calibrants,andthe first five sets of spots correspond to d -spacings (A˚)of 4.23,2.45,2.12,1.42and 1.23.More details are presented in Supplementary Information.Additionally,these high-resolution images show regions where fringes are observed and regions where they are not,which indicates that there is significant local curvature in thesheets.Figure 3|Electrical conductivity of the polystyrene–graphene composites as a function of filler volume fraction.Main figure,composite conductivity,j c ,plotted against filler volume fraction,f .Right inset,log j c plotted against log(f 2f c ),where f c is the percolation threshold (see text).Solid lines in both graphs are calculated conductivities based on the fitting (inset,log–log plot)of the experimental data to the effective conductivity equation described in the text.Fitted parameters are:t ¼2.74^0.20,j f ¼104.92^0.52S m 21and f c ¼0.1vol.%.Left inset:top and middle diagrams show the four-probe setup for in-plane and transverse measurements,respectively;bottom diagram,one of the computeddistributions of the current density (contour lines)with local directions and magnitude (shown by arrows)in a specimen for the following conditions —the sample thickness is twice the electrode width and the gap between them,and the in-plane resistivity is 10times lower than the transverse resistivity.LETTERSNATURE |Vol 442|20July 2006percolation threshold of around0.1vol.%is almost the same as was achieved for single-wall carbon nanotube(SWNT)–polystyrene composites made by latex technology27,and just twice and four times higher than those measured for SWNT–polyimide11,24and poly(phenyleneethynylene)-functionalized SWNT–polystyrene composites28,respectively.Absolute conductivities of our gra-phene–polystyrene composites are also essentially the same as the values reported for SWNT-filled polystyrene composites27.However, in contrast to these reports,which feature thinfilm composites,our samples are made by a quasi-industrial method(hot-pressing;we have also made samples by injection moulding that show similar values of electrical conductivity).Furthermore,graphene sheets have higher surface-to-volume ratios than SWNTs owing to the inaccessi-bility of the inner nanotube surface to polymer molecules.This makes graphene sheets potentially more favourable for altering all matrix properties—such as the mechanical,rheological and per-meability properties,and degradation stability.Also,SWNTs are still much more expensive than graphite,which is a commodity material with about1,000,000t used annually,and which is sold for a few US dollars per kilogram29.In contrast,graphite oxide can be readily prepared from a vast array of graphites,and its industrial production has also recently become a topic of interest30.We therefore expect that our method for preparation and incorpo-ration of individual graphene sheets into polymer matrices will lead to the further development of a broad new class of materials with enhanced properties and even introduce new functionalities to polymer composites.METHODSFabrication of composites.Graphite oxide was prepared by the Hummers method from SP-1graphite(Bay Carbon),and dried for a week over phosphorus pentoxide in a vacuum desiccator.Dried graphite oxide(50mg)was suspended in anhydrous DMF(5ml,Dow-Grubbs solvent system),treated with phenyl isocyanate(2mmol,Sigma-Aldrich)for24h,and recovered byfiltration through a sintered glass funnel(50ml,medium porosity).Stable dispersions of the resulting phenyl isocyanate-treated graphite oxide materials were prepared by ultrasonic exfoliation(Fisher Scientific FS60,150W,1h)in DMF (1mg ml21).Polystyrene(Scientific Polymer Products,approximate M w¼280kD,PDI¼3.0)was added to these dispersions and dissolved with stirring(Fig.1d,left).Reduction of the dispersed material(Fig.1d,right)was carried out with dimethylhydrazine(0.1ml in10ml of DMF,Sigma-Aldrich)at 808C for24h.Upon completion,the coagulation of the polymer composites was accomplished by adding the DMF solutions dropwise into a large volume of vigorously stirred methanol(10:1with respect to the volume of DMF used).The coagulated composite powder(Fig.1e)was isolated viafiltration;washed with methanol(200ml);dried at1308C under vacuum for,10h to remove residual solvent,anti-solvent,and moisture;crushed into afine powder with a mortar and pestle,and then pressed(Fig.1f)in a hydraulic hot press(Model0230C-X1, PHI-Tulip)at18kN with a temperature of2108C.Further description of the composite samples’preparation is given in Supplementary Information. To convert wt%loading of graphene sheets in the composite samples to vol.% (as used in the text),a density for the phenyl isocyanate-treated graphite oxide sheets of2.2g cm23was assumed along with the known density of polystyrene, 1.05g posites of acrylonitrile-butadiene-styrene and styrene-butadiene rubbers(Sigma-Aldrich)were similarly prepared using the appropriate copolymers.Microscopy.AFM images were taken on an AutoProbe CP/MT scanning probe microscope(MultiTask;Veeco Instruments).Imaging was done in non-contact mode using a V-shaped‘Ultralever’probe B(Park Scientific Instruments,boron-doped Si with frequency f c¼78.6kHz,spring constants k¼2.0–3.8N m21, and nominal tip radius10nm).All images were collected under ambient conditions at50%relative humidity and238C with a scanning raster rate of 1Hz.Samples for AFM images were prepared by depositing dispersions of functionalized graphite oxide in DMF on a freshly cleaved mica surface(T ed Pella Inc.)and allowing them to dry in air.Electron micrographs were acquired using afield emission SEM(LEO1525) and a TEM(JEM-3010,JEOL,operated at300kV).Two SEM imaging con-ditions were used:‘surface imaging’operating at#1kV,and‘sub-surface imaging’at$5kV.Microtomed and castfilm samples were prepared for TEM.Pressed composites were microtomed to slices of40–60nm thickness (Leica Ultracut UCT,Reichert Inc.)and dropped onto standard TEM grids.Samples investigated in this way were0.24,1.44and2.4vol.%.A polystyrene control sample was also prepared and imaged in this way.Before imaging with TEM,SEM was used to verify that a sufficient amount of highly dispersed platelets were present.Cast-film samples were prepared to image graphene sheets ‘lyingflat’on the carbon supportfilm,with the beam incident along the[0001] direction of the graphene sheet.A small amount(,0.01mg)of un-pressed powder composite was dissolved in toluene(10ml)together with additional polystyrene(,0.02mg)as diluent.The solution was sonicated(Crest Ultrasonic Tru-Sweep175HT,45W,1h)before casting,producing a highly dilute solution that,when cast on a mica substrate,formed afilm approximately40nm thick,as measured by AFM.Electrical measurements.Hot-pressed composite samples having0.3–0.5mm thickness were cut into strips(1–2mm wide and15–20mm long).A thin‘skin’layer deficient in the phenyl isocyanate-treated graphite oxide platelets was removed by oxygen plasma etching(Plasma-Preen II-862,Plasmatic Systems; 2torr O2,1.5min,350W)to reduce the contact resistance between the metal leads and sample tested.After etching,a thin goldfilm(,25nm)was thermally deposited(BOC Edwards Auto306Evaporation System)on one side of the sample for‘longitudinal’measurements(gap between contacts is0.15mm),and on the top and bottom sides for‘transverse’measurements.The d.c.resistance of the composites was measured with a d.c.power supply(HP6612C),digital multimeter(HP34401A)and picoammeter(Keithley6485)using a standard four-probe technique with a threshold detection limit of0.1G Q.Received18January;accepted5June2006.1.Dresselhaus,M.S.&Dresselhaus,G.Intercalation compounds of graphite.Adv.Phys.51,1–-186(2002).2.Hirata,M.,Gotou,T.,Horiuchi,S.,Fujiwara,M.&Ohba,M.Thin-film particlesof graphite oxide1:High-yield synthesis andflexibility of the particles.Carbon 42,2929–-2937(2004).3.Yu,M.F.,Lourie,O.,Moloni,K.,Kelly,T.F.&Ruoff,R.S.Strength and breakingmechanism of multiwalled carbon nanotubes under tensile load.Science287, 637–-640(2000).4.Novoselov,K.S.et al.Electricfield effect in atomically thin carbonfilms.Science306,666–-669(2004).5.Novoselov,K.S.et al.Two-dimensional gas of massless Dirac fermions ingraphene.Nature438,197–-200(2005).6.Zhang,Y.,Tan,Y.-W.,Stormer,H.L.&Kim,P.Experimental observation of thequantum Hall effect and Berry’s phase in graphene.Nature438,201–-204(2005).7.Zhang,Y.,Small,J.P.,Amori,M.E.S.&Kim,P.Electricfield modulation ofgalvanomagnetic properties of mesoscopic graphite.Phys.Rev.Lett.94,176803 (2005).8.Berger,C.et al.Ultrathin epitaxial graphite:two-dimensional electron gasproperties and a route toward graphene-based nanoelectronics.J.Phys.Chem.B108,19912–-19916(2004).9.Stankovich,S.et al.Stable aqueous dispersions of graphitic nanoplatelets viathe reduction of exfoliated graphite oxide in the presence of poly(sodium4-styrenesulfonate).J.Mater.Chem.16,155–-158(2006).10.Stauffer,D.&Aharnoy,A.Introduction to Percolation Theory(Taylor&Francis,London,1991).11.Ounaies,Z.,Park,C.,Wise,K.E.,Siochi,E.J.&Harrison,J.S.Electricalproperties of single wall carbon nanotube reinforced polyimide composites.Compos.Sci.Technol.63,1637–-1646(2003).12.Chung,D.D.L.Electrical applications of carbon materials.J.Mater.Sci.39,2645–-2661(2004).13.He,H.,Klinowski,J.,Forster,M.&Lerf,A.A new structural model for graphiteoxide.Chem.Phys.Lett.287,53–-56(1998).14.Lerf,A.,He,H.,Forster,M.&Klinowski,J.Structure of graphite oxide revisited.J.Phys.Chem.B102,4477–-4482(1998).15.Hirata,M.,Gotou,T.&Ohba,M.Thin-film particles of graphite oxide2:Preliminary studies for internal micro fabrication of single particle andcarbonaceous electronic circuits.Carbon43,503–-510(2005).16.Szabo,T.,Szeri,A.&Dekany,posite graphitic nanolayers prepared byself-assembly betweenfinely dispersed graphite oxide and a cationic polymer.Carbon43,87–-94(2005).17.Kovtyukhova,N.I.et yer-by-layer assembly of ultrathin compositefilmsfrom micron-sized graphite oxide sheets and polycations.Chem.Mater.11,771–-778(1999).18.Kotov,N.A.,Dekany,I.&Fendler,J.H.Ultrathin graphite oxide-polyelectrolytecomposites prepared by self-assembly.Transition between conductive andnon-conductive states.Adv.Mater.8,637–-641(1996).19.Cassagneau,T.,Guerin,F.&Fendler,J.H.Preparation and characterization ofultrathinfilms layer-by-layer self-assembled from graphite oxide nanoplatelets and ngmuir16,7318–-7324(2000).20.Vaia,R.A.&Wagner,H.D.Framework for nanocomposites.Mater.Today7,32–-37(2004).21.Du,X.S.,Xiao,M.,Meng,Y.Z.&Hay,A.S.Novel synthesis of conductivepoly(arylene disulfide)/graphite nanocomposite.Synth.Met.143,129–-132(2004).NATURE|Vol442|20July2006LETTERS22.Liu,P.&Gong,K.Synthesis of polyaniline-intercalated graphite oxide by an insitu oxidative polymerization reaction.Carbon37,706–-707(1999).23.Stankovich,S.,Piner,R.D.,Nguyen,S.T.&Ruoff,R.S.Synthesis andexfoliation of isocyanate-treated graphene oxide nanoplatelets.Carbon(in the press).24.McLachlan,D.S.et al.AC and DC percolative conductivity of single wallcarbon nanotube polymer composites.J.Polym.Sci.B43,3273–-3287(2005).25.Chen,G.,Weng,W.,Wu,D.&Wu,C.PMMA/graphite nanosheets compositeand its conducting properties.Eur.Polym.J.39,2329–-2335(2003).26.Garboczi,E.J.,Snyder,K.A.,Douglas,J.F.&Thorpe,M.F.Geometricalpercolation threshold of overlapping ellipsoids.Phys.Rev.E52,819–-828(1995).27.Grossiord,N.,Loos,J.&Koning,C.E.Strategies for dispersing carbonnanotubes in highly viscous polymers.J.Mater.Chem.15,2349–-2352(2005).28.Ramasubramaniam,R.,Chen,J.&Liu,H.Homogeneous carbon nanotubepolymer composites for electrical applications.Appl.Phys.Lett.83,2928–-2930 (2003).29.Olson,GS Mineral Commodity Summaries.k /minerals/pubs/commodity/graphite/graphmcs06.pdf l(2006).30.Titelman,G.I.&Bron,S.Method of Manufacturing of Graphite Oxide(GO)P1391–-P1392(Research Disclosure500,IMI TAMI Institute for Research& Development Ltd.,Haifa,2005).Supplementary Information is linked to the online version of the paper at /nature.Acknowledgements SEM was done at the Electron Probe Instrumentation Centre at Northwestern University.This work was funded by the NASA University Research,Engineering and Technology Institute on Bio-Inspired Materials(BIMat)and by the NSF NIRT grant‘Nanostructured carbons from self-assembled block copolymer precursors:From synthesis and characterization to devices’.We appreciate J.A.Ibers and A.L.Ruoff for critically reading an early version of this manuscript.Author Information Reprints and permissions information is available at /reprintsandpermissions.The authors declare no competing financial interests.Correspondence and requests for materials should be addressed to R.S.R.(r-ruoff@)or S.T.N.(stn@).LETTERS NATURE|Vol442|20July2006。
详细 介绍石墨烯英文版
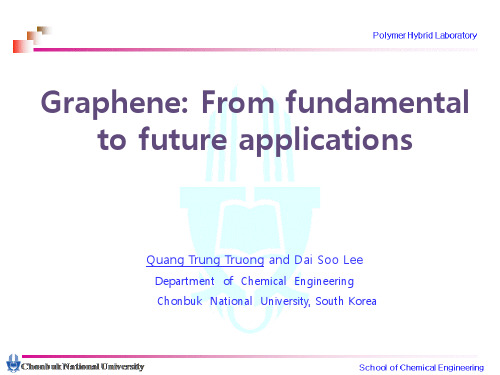
Notes Best electrical conductor of any known metal
59.6 × 106
Commonly used in electrical wire applications due to very good conductivity and price compared to silver.
Introduction
Properties of graphene
Mechanical properties
- High Young’s modulus (~1,100 Gpa) High fracture strength (125 Gpa)
- Graphene is as the strongest material
Content
Introduction to graphene. Preparation and characterization graphene Potential application of graphene Conclusions
Introduction to graphene
Graphene is a one-atom-thick planar sheet of sp2-bonded carbon atoms that are densely packed in a honeycomb crystal lattice The name ‘graphene’ comes from graphite + -ene = graphene
Molecular structure of graphene
High resolution transmission electron microscope images (TEM) of graphene
improve synthesis of graphene oxide

graphitic material,and indeed,either in thinfilms partial restoration of the graphitic structure accomplished by chemical reduction12,29Ϫ31to converted graphene(CCG).However,thestructure(with its desired properties)is not restored using these conditions,and significant de-introduced.32we reported the scalable preparation of oxide nanoribbons(GONRs)from multiwalled nanotubes by treatment with KMnO4and con-SO430and the discovery that the addition phosphoric acid(H3PO4)to this reaction produced more intact graphitic basal planes.33Re-these second-generation GONRs producedwere comparable in conductivity to thosereduction of thefirst-generation GONRs. hypothesized that this oxidation procedure(KMnO4 mixture of concentrated H SO/H PO,called gas evolution during preparation,and equivalent ductivity upon reduction,make it attractiveing material on a large scale.It may also show performance in materials applications,suchbranes,TEM grids,or temperature-sensitive fabrication.DISCUSSIONThe increased efficiency of the IGO methodpared to the HGO and HGOϩmethods wasafter thefirst purification step for each method. drophilic carbon material produced duringtion passed through the sieve,while the under-oxidized hydrophobic carbon material was retaineddue to its particle size and low water solubility.cantly less under-oxidized material was generatedthe production of IGO(0.7g)compared toor HGOϩ(3.9g)when starting with3g ofRepresentation of the procedures followed starting with graphiteflakes(GF).Under-oxidized hydrophobic recovered during the purification of IGO,HGO,and HGO؉.The increased efficiency of the IGO method small amount of under-oxidized material produced.techniques indicated that the order of HGOϽHGOϩϽIGO.Thermogravi-(TGA)of the materials(Figure4)showed losses between150and300°C,whichCO2,and steam release10from the functional groups.Between400and950°C,a slower mass loss was observed andto the removal of more stable oxygenBy TGA,HGO had the smallest weight and IGO had similar weight losses.Solid-state13C NMR(Figure5)suggests der of overall oxidation is HGOϽHGOspectra recorded using514nm laser excitation and(B)FTIR-ATR spectra of HGO؉Tapping mode AFM topographic images and height profiles of a single layer of(A)HGO؉,(B)HGO,and(C)can be assigned as described previously:10,35,36carbonyls near 190ppm;ester and lactol carbonyls 164ppm;graphitic sp 2carbons near 131ppm;ϪC(sp 3)ϪO near 101ppm;and alcohols at ppm with an upfield intense signal from epoxides 61ppm.The simplest measure of oxidation between the alcohol/epoxide signal and graphitic carbon signal.This ratio is greatest for IGO and HGO.It is also noteworthy that IGO appears to epoxide functionalities than either of the other X-ray diffraction (XRD)spectra (Figure 6)the same order of overall oxidation.For XRD,interlayer spacing of the materials is proportional degree of oxidation.The spacings are 9.5,9.0,and IGO,HGO ϩ,and HGO,respectively.Also,the spectrum had a peak at 3.7Å,indicating that traces starting material (graphite flakes)were present in O,286.2eV),carbonyl (C A O,287.8eV),and carbox-ylates (O ϪC A O,289.0eV).10All percentages of the dized materials were combined such that IGO had oxidized carbon and 31%graphitic carbon;HGO ϩand 37%;HGO contained 61and 39%of the oxi-dized carbon and graphitic carbon,respectively.The XPS spectra were then normalized with respect C A C peak (Figure 7).The degree of oxidation sample is similar to the amount indicated by NMR.IGO is the most oxidized material,HGO ϩisslightly (ϳ10%)less oxidized,and HGO is the least dized.Moreover,the apparent peak at ϳ287eV,corre-sponding to the oxidized carbons for IGO,is sharper the same peak for HGO ϩ.This suggests that,similar levels of overall oxidation,the IGO has a more regular structure than that of HGO ϩ.38Transmission electron microscopy (TEM)images three samples support the assertion that IGO has 4.TGA plots of HGO ؉,HGO,and IGO.Figure 5.13C NMR (50.3MHz)spectra obtained of HGO,HGO ؉and IGO [12kHz magic angle spinning (MAS),a 90°13C pulse,41ms FID,and 20s relaxation delay].Integration areas are shown under each peak.Figure 6.XRD spectra of HGO,HGO ؉,and IGO (1.54059␣1as wavelength).The UV/vis spectra of the three materials suggest the more ordered structure of IGO is due to greater retention of carbon rings in the basal planes.The spectra were recorded for an equal concentration material (Figure 9).The degree of remaining jugation can be determined by the max of each UV/vis spectrum.The more ¡*transitions (conjugation),less energy needs to be used for the electronic which results in a higher max .IGO,HGO ϩ,all have a very similar max ,which is in the231nm range as previously reported for GO.for all three materials,a similar shoulder around nm is observed and can be attributed to n ¡transitions of the carbonyl groups.This suggests materials are grossly similar in structure,as the IR,and AFM data indicated.However,IGO has extinction coefficient than those of HGO ϩsuggesting that,for an equal amount of each sample,the IGO has more aromatic rings or isolated Bulk samples of the oxidized materials were re-duced using hydrazine hydrate and then annealed and 900°C in Ar/H 2.In general,for the hydrazine duction,100mg of the IGO,HGO,or HGO ϩmaterial dispersed in 100mL of DI water,stirred for 30then 1.00mL of hydrazine hydrate was added.mixtures were heated at 95°C using a water min;a black solid precipitated from the reaction mixture.Products were isolated by filtration (PTFE pore size)and washed with DI water (50mL,methanol (20mL,3ϫ),producing 54,57,and of the reduced chemically converted IGO (CCIG),chemically converted HGO ϩ(CCHG ϩ),and chemically converted HGO (CCHG),respectively.After reduction,signal from oxidized carbons could be detected Only a broad aromatic/alkene NMR signal could detected for CCHG,CCHG ϩ,and CCIG.This signal shifted upfield relative to that in the precursor HGO ϩ,and IGO;the peak maximum after reduc-7.C1s XPS spectra of HGO ؉,HGO,and IGO normal-with respect to the C A C peak.images of (A)HGO ؉,(C)HGO,(E)IGO and their diffraction patterns (B),(D),and (F),respectively.electron diffraction (SAED)patterns corresponded to the graphene films in the TEM images.support films (Ted Pella,Inc.)were used to prepare the samples.Figure 9.UV/vis spectra recorded in aqueous solutions mg/mL of HGO ؉,HGO,and IGO.the tuning and matching of the13C and1H channels of the probe.After reduction and annealing in Ar/H2at900°C,no NMR signal could be detected for any of the samples,consistent with their becoming even more graphite-like.35XPS analysis of the black solids showed similar lev-els of reduction for all three materials when they were hydrazine reduced and when they were annealed(Fig-ure10;in Figure10B,C,the annealed materials are des-ignated by the prefix“ann”to differentiate them from standard e-beam lithography(we used PMMA as a posi-tive resist),and then20nm thick Pt contacts were formed by e-beam evaporation and lift-off process.Fig-ure11C shows a SEM image of a typical electronic device.The electrical measurements were performed using a probe station(Desert Cryogenics TT-probe6system) under vacuum with chamber base pressure below10Ϫ5 Torr.Normally,the devices were kept under vacuum for at least2days before the measurements.TheFigure10.C1s XPS spectra of(A)hydrazine reduced,(B)further300°C/H2-annealed materials,(C)900°C/H2-annealed materials. The annealed materials in panels B and C are designated by the prefix“ann”to differentiate them from the materials in A that were only hydrazine reduced.annealed in Ar/H2at300or900°C for0.5h.Forthermally annealed materials,we measured atdevices for each material.We found that,after thermal treatment at300°C,the conductivities ofannCCG materials dramatically increase up to2.1Ϯ(annCCHG),6.8Ϯ4.4S/cm(annCCHGϩ),andS/cm(annCCIG);the scatter plot is shown inThe annCCHG is statistically significantly less conductive than the other two samples(pϽ0.05,t assuming equal variance),but due to the smalldevices for annCCHGϩ,it is difficult to deter-annCCHGϩand annCCIG are significantlyThe statistical significance of the increase in conductivity for annCCHGϩcompared to annCCIG is pϭ0.04,t test assuming equal variance)and completely driven by the outlier point at15.0(remov-point,pϭ0.24,t test assuming equal variance).well as in other cases,the conductivities were calculated assuming the thicknesses of theflakesG materials annealed at even higherture of900°C exhibit further increases in conductivities up to375Ϯ215S/cm(annCCHG),350Ϯ125 (annCCHGϩ),and400Ϯ220S/cm(annCCIG)11F).There is no statistically significant difference tween any of the samples after annealing at900 gesting that high temperature annealing eliminates most of the differences in composition and structure present in the three samples.CONCLUSIONSThe improved method for producing GOcant advantages over Hummers’method.Thefor running the reaction does not involve a large therm and produces no toxic gas.Moreover,proved method yields a higher fraction of well-oxidized hydrophilic carbon material.This IGO is more(A,B)SEM images of CCIGflakes on Si/SiO2substrate.(C)SEM image of a typical electronic device for CCIGflake.(D)Source؊drain current(I sd)؊gate voltage(V g)characteristics of the same electronic device CCIGflake measured in air and in vacuum after3days of evacuation in the probe station chamber at ؊5Torr.(E)Plot of the mean conductivities for the monolayer annCCHG,annCCHG؉,and annCCIGflakesAr/H2at300°C for0.5h.(F)Plot of the mean conductivities for the monolayer annCCHG,annCCHG؉, annealed in Ar/H2at900°C for0.5h.。
TMG生产石墨烯

Triton Minerals LtdASX:ABN:Street address:Postal address:Tel:Fax:Email:**************************.au Web:.auProjects:Balama NorthAncuabeBalama SouthTMG PRODUCES GRAPHENEHIGHLIGHTS∙Trial production of Graphene Oxide completed∙Graphene Oxide readily produced from different graphite concentrate grades∙Commercial grade Graphene Oxide produced∙Graphene successfully produced∙TMG product range expandedTriton Minerals Limited(ASX: TON, Triton or Company) is pleased to announce the positive initial Graphene Oxide tests conducted on the TMG concentrates.Triton’s Managing Director & CEO Brad Boyle said: “The successful production of the Graphene Oxide and Graphene through standard industrial techniques, once again demonstrates the high quality and versatility of the TMG products.The remarkable properties of Graphene are well known and now that TMG has shown to be ideally suited to create the wonder material, this now creates further possibilities for Triton to expand their market presence and to explore an even broader client base and revenue streams.”GRAPHENE OXIDEIn tests conducted by a Government-sponsored laboratory in Singapore, Graphene Oxide has been successfully produced from various graphite concentrate grades of TMG product.Commercial-grade Graphene Oxide was readily produced from a broad range of TMG concentrates, using the standard extraction methods. These initial results are considered by Triton to be encouraging.In these industry-standard production runs only 1.5 grams of TMG concentrate was required to produce approximately 250ml of high concentration graphene oxide solution.Research has found that graphene oxide solution is sold commercially in a diluted form (concentration at 4mg/ml) and can sell for of up to $400 for 250ml. Triton considers that the economics of producing graphene oxideand subsequently graphene from TMG are very encouraging.The Company will continue to explore opportunities to refine the production of Graphene Oxide. As the predicted global demand for graphene oxide and graphene grows, Triton is well positioned to help meet that supply demand, with the ability to produce high purity and volume of graphite concentrate.Figure 1. Image of the Graphene Oxide solution (left) created from TMG concentrates. SEM Imaging of fine Graphene Oxide flakes (right) created from TMG concentrate. The size of the flakes ranged from approximately 100 nm to 3 μm.GRAPHENETriton confirms the same Singapore laboratory has also successfully created Graphene powder from the TMG products by simply reducing (drying) the TMG Graphene Oxide solution. Once the Graphene powder has been created it is actually insoluble in water.WHAT IS GRAPHENE OXIDEGraphene oxide (GO) is a unique material that can be viewed as a single monomolecular layer of graphite with various oxygen containing functionalities.Interest in GO has increased dramatically after graphene, a single layer of graphite, was first isolated and studied.Reduced GO can be made as a thin film from an aqueous dispersion of GO in water and has moderate conductivity, it is attractive for use in electronic devices. In addition to being components in electronic devices, GO and reduced GO have been used in nanocomposite materials, polymer composite materials, energy storage,biomedical applications, catalysis,and as a surfactant with some overlaps between these fields (Source: ).Figure 3. Graphene oxide (concentration level at 4 mg/mL, dispersion in H2O) PROPERTIES OF GRAPHENE OXIDEGraphene oxide is its easy dispersability in water and other organic solvents, as well as in different matrixes, due to the presence of the oxygen. The is a very important property when mixing the material with ceramic or polymer matrixes when trying to improve their electrical and mechanical properties. However, on the other hand, the electrical conductivity of GO is reduced and sometimes described as an electrical insulator, due to the bonding of the oxygen molecules (Source: ).There are many ways in which GO can be functionalized, depending on the desired application. For optoelectronics, biodevices or as a drug-delivery material, for example, it is possible to substitute amines for the organic covalent functionalization of graphene to increase the dispersability of chemically modified graphenes in organic solvents. It has also been proved that porphyrin-functionalized primary amines and fullerene-functionalized secondary amines could be attached to graphene oxide platelets, ultimately increasing nonlinear optical performance (Source: ).In order for GO to be usable as an intermediary in the creation of monolayer or few-layer graphene sheets, it is important to develop an oxidization and reduction process that is able to separate individual carbon layers and then isolate them without modifying their structure.GRAPHENE OXIDE APPLICATIONSThere are many applications which use GO and the list continues to grow. There are many obstacles needed to be overcome before GO can be used commercially in most areas but advancements are being made every day and some of the key areas of development for GO include bioengineering, composite materials, energy technology and nanotechnology.Energy StorageNanocomposites of reduced GO have been used for high capacity energy storage in lithium ion batteries. In these studies, electrically insulating metal oxide nanoparticles were adsorbed onto reduced GO to increase the performance of these materials in batteries. High surface area of reduced GO has been made using microwaves for exfoliation and reduction of GO. The high surface area reduced GO formed is useful as an energy storage material in super capacitors (Source: ).Graphene based micro-supercapacitors will likely be developed for use in low energy applications such as smart phones and portable computing devices. Graphene-enhanced lithium ion batteries could be used in much higher energy usage applications such as electrically powered vehicles, or they can be used as lithium ion batteries are now, in smartphones, laptops and tablet PCs but at significantly lower levels of size and weight (Source: ).Photovoltaic CellsOffering very low levels of light absorption (at around 2.7% of white light) whilst also offering high electron mobility means that graphene can be used as an alternative to silicon or ITO in the manufacture of photovoltaic cells. Silicon is currently widely used in the production of photovoltaic cells, but while silicon cells are very expensive to produce, graphene based cells are potentially much less so. When materials such as silicon turn light into electricity it produces a photon for every electron produced, meaning that a lot of potential energy is lost as heat. Recently published research has proved that when graphene absorbs a photon, it actually generates multiple electrons. Also, while silicon is able to generate electricity from certain wavelength bands of light, graphene is able to work on all wavelengths, meaning that graphene has the potential to be as efficient as, if not more efficient than silicon, ITO or (also widely used) gallium arsenide. Being flexible and thin means that graphene based photovoltaic cells could be used in clothing; to help recharge your mobile phone, or even used as retro-fitted photovoltaic window screens or curtains to help power your home (Source: ).ElectronicsIt is predicted that Optoelectronics; specifically touchscreens, liquid crystal displays (LCD) and organic light emitting diodes (OLEDs) will soon be using GO in a commercial volumes (Source: ). Other Electronic devices using GO as a starting material for at least one of the key components, including the field effect transistor (FET) that uses reduced GO as a chemical sensor and biosensor to detect hormonal catecholamine molecules, avidin and DNA. Whilst, another study had GO functionalised with glucose oxidase and deposited on an electrode was used as an electrochemical glucose sensor. (Source: ).UltrafiltrationAnother standout property of graphene is that while it allows water to pass through it, it is almost completely impervious to liquids and gases (even relatively small helium molecules). This means that graphene could be used as an ultrafiltration medium to act as a barrier between two substances. The benefit of using graphene is that it is only 1 single atom thick and can also be developed as a barrier that electronically measures strain and pressures between the 2 substances.This could mean that graphene is developed to be used in water filtration systems, desalination systems and efficient and economically more viable biofuel creation (Source: ).Composite MaterialsGraphene is strong, stiff and very light. Currently, aerospace engineers are incorporating carbon fibre into the production of aircraft as it is also very strong and light. However, graphene is much stronger whilst being also much lighter. Ultimately it is expected that graphene is utilized to create a material that can replace steel in the structure of aircraft, improving fuel efficiency, range and reducing weight. Due to its electrical conductivity, it could even be used to coat aircraft surface material to prevent electrical damage resulting from lightning strikes. In this example, the same graphene coating could also be used to measure strain rate, notifying the pilot of any changes in the stress levels that the aircraft wings are under. These characteristics can also help in the development of high strength requirement applications such as body armour for military personnel and vehicles (Source: ).Biomedical ApplicationsGO has been used as a component in drug delivery systems. Functionalized nanographene oxide has been used in several studies on targeted delivery of anti-cancer drugs (Source: ).BiosensorsGO and reduced GO have been used as components in several systems designed to detect biologically relevant molecules. Folic acid functionalized GO was used as a component in a system used to detect human cervical cancer and human breast cancer cells (Source: ).CONVERTING GRAPHITE TO GRAPHENE OXIDETriton confirms that there are many ways being developed by companies around the world to produce commercial volumes of GO and graphene, however, at the moment the most popular method to produce graphene is by using mechanical or thermal exfoliation, chemical vapour deposition (CVD), and epitaxial growth.Many modern procedures for the synthesis of GO are based on the method first reported by Hummers in which graphite is oxidized by a solution of potassium permanganate in sulphuric acid. Reduction of GO has been reported using hydrazine, NaBH4, ascorbic acid, and HI. GO can be reduced as a thin film or in an aqueous solution (Source: ).It appears the most effective way of synthesised graphene on a large scale is through chemical reduction of graphene oxide. However, it has been difficult for scientists to complete the task of producing graphene sheets of the same quality as mechanical exfoliation (Source: ). Interest in GO continues to grow as people search for a cheaper, simpler, more efficient and better yielding method of producing graphene, that can be scaled up massively compared to current methods, and be financially suitable for industrial or commercial applications. Once this issue is overcome, we can expect to see graphene become much more widely used in commercial and industrial applications (Source: ).CONCLUSIONS – What does this mean for Triton?The demand and use of Graphene Oxide and Graphene continues to grow rapidly around the world in areas of bioengineering, composite materials, energy technology and nanotechnology. Triton has successfully produced Graphene Oxide and Graphene, using industry-standard methods, from the TMG concentrate.This further demonstrates the high quality and versatility of the TMG concentrate products and creates further possibilities for Triton to expand their market presence and to explore a broader customer and application base and revenue stream.Triton is rapidly working towards establishing TMG as a new global graphite-industry benchmark, by aiming to offer the world's lowest cost and most diversified graphite product range together with the longevity of a reliable supply of high quality flake graphite.RegardsBrad BoyleCEO & Managing DirectorTriton Minerals LtdHolder of the world’s largest known combined graphite-vanadium resourceVisionLed by a highly experienced Board and Management team, Triton’s primary vision is to grow shareholders value through discovery and development of graphite, gold and other precious, base and industrial minerals deposits. Further, Triton will explore vertical integration opportunities to supplement its core business and to create valued revenue streams to ultimately benefit Triton’s shareholders.TMG and beyondTriton hopes to establish Triton Mozambique graphite, produced from its Mozambique graphite projects (TMG) as the global graphite-industry benchmark by aiming to offer the world’s lowest cost and most diversified graphite product range, together with the longevity of a reliable supply of high quality flake graphite.Triton hopes to establish Triton Mozambique graphite, produced from its Mozambique graphite projects (TMG) as the global graphite-industry benchmark.Triton is also actively pursuing vertical integration opportunities to be involved in all aspects of the graphite supply chain, which Triton believes will add significant value to the Company and its shareholders in the long term.For further information, please contact:Brad BoyleCEO & Managing DirectorTel: + 61 8 6489 2555Email: ****************************.auForward-Looking StatementsThis document may include forward-looking statements. Forward-looking statements include, but are not necessarily limited to, statements concerning Triton Minerals Limited’s planned exploration program and other statements that are not historic facts. When used in this document, th e words such as “could”, “plan”, “estimate” “expect”, “intend”, “may”, “potential”, “should” and similar expressions are forward-looking statements. Although Triton Minerals Limited believes that its expectations reflected in these are reasonable, such statements involve risks and uncertainties, and no assurance can be given that actual results will be consistent with these forward-looking statements.。
基于分子动力学的氧化石墨烯对PVA纤维-CSH界面影响机理研究
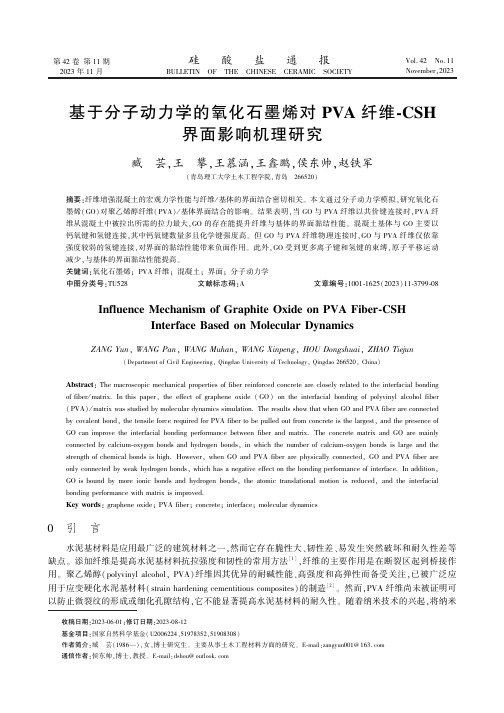
第42卷第11期2023年11月硅㊀酸㊀盐㊀通㊀报BULLETIN OF THE CHINESE CERAMIC SOCIETY Vol.42㊀No.11November,2023基于分子动力学的氧化石墨烯对PVA 纤维-CSH界面影响机理研究臧㊀芸,王㊀攀,王慕涵,王鑫鹏,侯东帅,赵铁军(青岛理工大学土木工程学院,青岛㊀266520)摘要:纤维增强混凝土的宏观力学性能与纤维/基体的界面结合密切相关㊂本文通过分子动力学模拟,研究氧化石墨烯(GO)对聚乙烯醇纤维(PVA)/基体界面结合的影响㊂结果表明,当GO 与PVA 纤维以共价键连接时,PVA 纤维从混凝土中被拉出所需的拉力最大,GO 的存在能提升纤维与基体的界面黏结性能㊂混凝土基体与GO 主要以钙氧键和氢键连接,其中钙氧键数量多且化学键强度高㊂但GO 与PVA 纤维物理连接时,GO 与PVA 纤维仅依靠强度较弱的氢键连接,对界面的黏结性能带来负面作用㊂此外,GO 受到更多离子键和氢键的束缚,原子平移运动减少,与基体的界面黏结性能提高㊂关键词:氧化石墨烯;PVA 纤维;混凝土;界面;分子动力学中图分类号:TU528㊀㊀文献标志码:A ㊀㊀文章编号:1001-1625(2023)11-3799-08Influence Mechanism of Graphite Oxide on PVA Fiber-CSH Interface Based on Molecular DynamicsZANG Yun ,WANG Pan ,WANG Muhan ,WANG Xinpeng ,HOU Dongshuai ,ZHAO Tiejun (Department of Civil Engineering,Qingdao University of Technology,Qingdao 266520,China)Abstract :The macroscopic mechanical properties of fiber reinforced concrete are closely related to the interfacial bonding of fiber /matrix.In this paper,the effect of graphene oxide (GO)on the interfacial bonding of polyvinyl alcohol fiber (PVA)/matrix was studied by molecular dynamics simulation.The results show that when GO and PVA fiber are connected by covalent bond,the tensile force required for PVA fiber to be pulled out from concrete is the largest,and the presence of GO can improve the interfacial bonding performance between fiber and matrix.The concrete matrix and GO are mainly connected by calcium-oxygen bonds and hydrogen bonds,in which the number of calcium-oxygen bonds is large and the strength of chemical bonds is high.However,when GO and PVA fiber are physically connected,GO and PVA fiber are only connected by weak hydrogen bonds,which has a negative effect on the bonding performance of interface.In addition,GO is bound by more ionic bonds and hydrogen bonds,the atomic translational motion is reduced,and the interfacial bonding performance with matrix is improved.Key words :graphene oxide;PVA fiber;concrete;interface;molecular dynamics 收稿日期:2023-06-01;修订日期:2023-08-12基金项目:国家自然科学基金(U2006224,51978352,51908308)作者简介:臧㊀芸(1986 ),女,博士研究生㊂主要从事土木工程材料方面的研究㊂E-mail:zangyun001@通信作者:侯东帅,博士,教授㊂E-mail:dshou@ 0㊀引㊀言水泥基材料是应用最广泛的建筑材料之一,然而它存在脆性大㊁韧性差㊁易发生突然破坏和耐久性差等缺点㊂添加纤维是提高水泥基材料抗拉强度和韧性的常用方法[1],纤维的主要作用是在断裂区起到桥接作用㊂聚乙烯醇(polyvinyl alcohol,PVA)纤维因其优异的耐碱性能㊁高强度和高弹性而备受关注,已被广泛应用于应变硬化水泥基材料(strain hardening cementitious composites)的制造[2]㊂然而,PVA 纤维尚未被证明可以防止微裂纹的形成或细化孔隙结构,它不能显著提高水泥基材料的耐久性㊂随着纳米技术的兴起,将纳米3800㊀水泥混凝土硅酸盐通报㊀㊀㊀㊀㊀㊀第42卷材料(如碳纳米管(carbon nanotubes,CNTs)[3-5]㊁石墨烯和氧化石墨烯(graphene oxide,GO)[6-8])应用于水泥基材料来提高性能已成为近年来新的研究热点㊂与疏水碳纳米管和石墨烯不同,氧化石墨烯引入了含氧基团,如羟基㊁羰基和羧基,是一种优秀的亲水材料[9-10]㊂研究表明,氧化石墨烯的加入可以提高混凝土的抗压抗折强度[11]和耐久性[12-14]㊂因此,利用PVA纤维和氧化石墨烯的协同效应,有望大幅提高水泥基材料的综合性能㊂李相国等[15]研究证明GO复掺PVA纤维可以显著改善水泥基材料孔结构,降低孔隙率,提高水泥基材料抗氯离子渗透性能并降低水泥基材料收缩率㊂Jiang等[16]研究发现在水泥基材料中添加氧化石墨烯可以起到细化孔隙结构的作用,同时提高水化产物在PVA纤维上的黏附性,有利于PVA纤维与水泥基体的结合㊂氧化石墨烯与PVA纤维耦合改性的水泥基材料表现出优异的力学强度和耐久性㊂Yao等[17]利用氧化石墨烯对PVA纤维表面改性,使其化学键能提高80倍以上,混凝土试样抗拉强度提高35.6%㊂然而,GO的存在打破了原有的界面结构,纤维与基体之间的界面变成纤维与GO之间的界面和GO与基体间的界面,界面中化学键的键合也发生了改变㊂在纤维增强水泥基复合材料中,纤维和基体分担载荷,应力通过纤维和基体间的界面传递㊂因此,界面的组成和性能是决定纤维增强水泥基复合材料性能的关键㊂Wang等[18]通过分子动力学分析发现,PVA纤维中的羟基与水化产物中的钙离子可以形成稳定的离子键,因此PVA纤维与水化产物有较好的黏结力㊂GO对界面的影响尚不清晰,而这将直接影响纤维增强水泥基材料的性能㊂本文先通过拉拔模拟计算纤维从界面中拉出所需要的拉拔力,借助力学响应描述界面黏附行为㊂通过静态结构和动态结构分析,探究GO对PVA/CSH界面黏结性能的影响机理㊂本研究有助于指导GO和PVA 在水泥基复合材料中发挥最佳的协同作用㊂1㊀模拟方法1.1㊀模型建立依据Pellenq等[19]和Manzano等[20]提出的方法,基于托贝莫来石(11Å)结构构建了Ca/Si比值为1.7的水化硅酸钙(CSH)模型㊂选取无水托贝莫来石(11Å)层状结构作为建模的初始构型㊂根据硅链的Q n分布结果,随机删除桥接的SiO2和二聚体结构(Si2O4),得到复合硅链的钙硅骨架和层状结构㊂再通过巨正则蒙特卡洛法(GCMC)模拟水分子逐渐吸附在钙硅骨架孔隙上直至饱和这一过程㊂根据液态水在室温下的性质,设定化学势为0,温度为300K,成功建立CSH初始模型,如图1(a)所示㊂根据Allington等[21]提出的方法构建氧化石墨烯模型㊂将石墨烯的单位单元(x=4.62Å,y=3.69Å,z=3.40Å,α=β=γ=90ʎ)在x和y 方向分别扩大,再将环氧( O )㊁羟基( OH)和羧基( COOH)三个官能团修饰到石墨烯上㊂其中 O 和 OH基团分布在石墨烯表面, COOH基团分布在石墨烯边缘,如图1(b)所示㊂氧化石墨烯薄片的化学成分为C10O1(OH)1(COOH)0.5,符合氧化石墨烯典型官能团覆盖率,即含氧量为20%~30%[22]㊂PVA纤维模型如图1(c)所示,每个PVA纤维链具有50个碳原子㊂参照文献[23]结果,本文建立了两种GO/PVA模型:第一种PVA模型如图1(d)所示,GO物理附着在PVA表面,无共价键连接;第二种PVA模型如图1(e)所示,GO与PVA纤维通过脱水缩聚,形成酯基 COO ,使GO与PVA纤维以共价键形式连接㊂因模型中含有多种氧原子和氢原子,为了方便后面的分析将其进行区分㊂O s表示CSH中硅氧四面体中的非桥接氧原子,O hs表示CSH的羟基氧原子,O hp表示PVA纤维中的氧原子,O o表示GO片中C O C中的氧原子, O hg表示GO片中 OH中的氧原子㊂此外, COOH中有两种位置的氧原子,O 表示以双键连接碳的氧原子,O hg表示以单键连接碳的氧原子㊂H op表示PVA纤维中的羟基氢原子,H og表示GO片中含氧官能团中的氢原子㊂从所建立的CSH分子模型层间处切开,并向外平移以预留足够空间插入GO和PVA纤维,并在y方向预留足够的空间保证纤维可以拉出㊂将PVA纤维插入CSH形成的纤维界面定义为界面CP,如图1(f)所示㊂将PVA/GO(物理附着)插入CSH,纤维与GO形成的界面定义为界面CGP1,如图1(g)所示㊂将PVA-GO(形成共价键)插入CSH,纤维与GO成为一体后与CSH形成的界面定义为界面CGP2,如图1(h)所示㊂三个模拟盒子尺寸大小一致,均为x=44.10Å,y=160.70Å,z=67.47Å,α=β=γ=90ʎ㊂㊀第11期臧㊀芸等:基于分子动力学的氧化石墨烯对PVA纤维-CSH界面影响机理研究3801图1㊀氧化石墨烯㊁PVA纤维㊁CSH的结构及连接方式Fig.1㊀Structures and connection modes of graphene oxide,PVA fiber and CSH1.2㊀力㊀场力场的选择对模拟的准确性十分关键,CSH基底采用CLAYFF力场,此力场已被成功用于黏土㊁水泥水化产物㊁多组分矿物体系的模拟[24-25]㊂PVA纤维采用CVFF力场,CVFF已被广泛应用于有机物建模[26-27]㊂CLAYFF与CVFF联合力场已证明适用于模拟胶凝材料与聚合物㊁有机物之间的界面特性[28]㊂此联合力场3802㊀水泥混凝土硅酸盐通报㊀㊀㊀㊀㊀㊀第42卷采用Lorentz-Berthelot 混合规则[29]㊂1.3㊀模拟过程模拟过程分为预平衡阶段㊁平衡阶段和拉拔阶段㊂所有模拟均采用大尺度原子/分子大规模并行模拟器(LAMMPS)平台进行计算㊂所有原子在NPT 系统中弛豫1000ps,时间步长设置为1fs,温度为300K㊂平衡后再运行1000ps,此阶段保持系统与环境变量均不变,每1ps 记录一次轨迹数据,收集到1000帧原子轨迹,用于平衡状态下界面结构和动力学行为分析㊂此后,在NVT 系综下对已经平衡的模型进行拉拔模拟,每根纤维链最右侧的碳原子作为外力的位置[30],时间步长设置为1fs㊂外力和界面能每100s 记录一次㊂拉拔力计算如式(1)所示㊂F =K [(x 0+vt )-x com ](1)式中:F 为拉拔力,x 0为所选碳原子在纤维y 方向上的初始质心,K 为弹簧常数,v 为拉力的速度,t 为模拟时间,x com 为所选碳原子质心沿y 方向的动态位置㊂2㊀结果与讨论2.1㊀拉拔过程图2㊀三个界面的拉力-时间关系Fig.2㊀Pull-out force-time relations of three interfaces 通过拉拔模拟计算得到拉拔力-时间曲线,如图2所示㊂在没有GO 的情况下纤维与CSH 形成界面CP㊂加入GO 后,纤维与GO 形成了新的界面CP1㊂从图2中可以清楚地看到,PVA 纤维从CSH 中被拉出所需要的拉力明显高于PVA /GO 从CSH 中被拉出所需要的拉力,这说明PVA /CSH 的界面黏结力优于PVA /GO界面的黏结力㊂此外,三个界面的拉拔力均波动较大,呈锯齿形逐渐减小㊂在模拟初期,拉拔力逐渐增大,主要原因是化学键的伸长㊂当化学键被过度拉伸直至断裂,拉力会突然下降,在松弛阶段又形成新的化学键,导致拉力再次增大,因此拉拔力呈波动状态㊂随着纤维拉出长度增加,界面处化学键减少,拉拔力逐渐减小㊂由此得出,纤维的拔出过程伴随化学键的断裂和重组㊂图3为拉拔过程第1000ps 快照,从图3(a)中可以发现,GO 与PVA 以物理方式连接时,纤维会从GO 之间很轻易地被拉出,说明GO /PVA 界面的黏结性能很差,GO 的加入对界面的黏结性能起到消极作用㊂如图3(b)所示,在PVA 纤维和GO 以共价键形式连接时,PVA 纤维和GO 作为一个整体同时被拉出㊂PVA-GO 与CSH 形成界面CGP2,此时纤维被拉出所需要的拉力最大,说明CGP2界面的黏结性能最好,GO 的加入对界面的黏结性能起到增强作用㊂图3㊀拉拔过程第1000ps 快照Fig.3㊀Snapshots of pull-out process at 1000ps㊀第11期臧㊀芸等:基于分子动力学的氧化石墨烯对PVA纤维-CSH界面影响机理研究3803 2.2㊀界面静态结构分析径向分布函数(radial distribution function,RDF)表示周围原子出现在中心原子一定范围内的可能性,是原子局部密度与系统的平均密度比值㊂RDF曲线可定性表征配位原子之间的空间相关性,曲线出现峰值的距离越近,表明两原子之间排列更规则,形成的化学键更强,峰值越高说明两原子相关性更高㊂同时,RDF也揭示了界面的连接机制㊂图4为径向分布函数计算结果㊂在CP界面中Ca O hp和氢键的RDF曲线均存在明显的峰值,说明在CSH与PVA纤维之间既存在Ca O hp离子键连接也存在氢键连接,如图4(a)所示㊂而在CP1界面(GO与PVA纤维之间),GO中的氧与PVA纤维中的氢形成脆弱的氢键,如图4(b)所示㊂与氢键相比,离子键的键能远大于氢键㊂键能越大,化学键越牢固,含有该键的分子越稳定,这充分说明了CSH与PVA纤维之间的联系远强于GO与PVA纤维的联系㊂图4㊀CGP1界面㊁CGP2界面和CP界面的径向分布函数Fig.4㊀RDF of CGP1,CGP2and CP interfaces在PVA纤维与GO以共价键连接时,PVA纤维与GO形成一个整体,与CSH之间形成了界面CGP2㊂Ca O o㊁Ca O hg㊁Ca O RDF曲线均存在明显的峰值,如图4(c)所示,这说明GO中的含氧官能团均可以与CSH中的钙离子形成离子键,钙离子与GO中氧原子连接,充当了GO与CSH之间的桥梁㊂其中Ca O hg 和Ca O o的RDF曲线峰值更高,说明离子键中的氧位主要由 OH和 O 提供㊂与CP界面相比,CGP2界面中的Ca O的RDF曲线峰值更高,这是因为在GO中拥有更多能够与CSH中阳离子形成有效化学键的极性位点㊂除了离子键外,界面CP和CGP2中氢键的RDF曲线都有明显的峰值,但在CGP2界面中氢键RDF曲线位置更为靠左,峰值更尖锐,这充分说明在这个界面中有更强的界面成键相互作用,如图4(d)所示㊂根据以上分析可以得出结论,在水泥基质中掺入的GO片可以通过离子键和氢键连接硅酸盐骨架,从而加强了有机相和无机相之间的界面连接㊂在拉拔模拟阶段,纤维从CSH中被拉出的过程中,大量的化学键会被拉伸甚至断裂㊂键长越短,原子核之间的距离越小,键能越大,化学键越稳定,破坏这种化学键需要克服的能量就越多㊂化学键越稳定,对原子3804㊀水泥混凝土硅酸盐通报㊀㊀㊀㊀㊀㊀第42卷运动的阻碍就越大,化学键越难断裂㊂根据前面的分析,在CGP2界面中形成的Ca O离子键和氢键数量最多,稳定性高,这意味着需要克服很大的键能才能将纤维从CSH中拉出㊂而PVA纤维与GO之间只有较弱的氢键,键能很小,较小的拉力就能将纤维拉出㊂拉拔仿真结果与本节分析结果一致㊂键合结构图如图5所示㊂图5㊀界面键合示意图Fig.5㊀Interface bonding diagrams化学键的稳定性可以通过时间相关函数(temporal correlation function,TCF)来描述㊂TCF值接近1,表明化学键稳定㊂如果TCF的值降至零,则说明化学键易断开且不稳定㊂根据前面章节的分析可知,CP界面和CGP2界面是通过Ca O离子键和氢键连接,而CGP1界面仅仅通过氢键将PVA纤维与CSH连接㊂本小节计算了三种界面间的化学键的时间相关函数,计算结果如图6(a)所示㊂在CGP2界面中Ca O键的TCF 值略高于CP界面,这意味着PVA和CSH之间形成的化学键更频繁地断裂和重组㊂在CGP2界面中, Ca O 键㊁Ca O o键和Ca O hg键的TCF值分别稳定在0.80㊁0.90和0.98左右,略有波动㊂与Ca O hg键和Ca O o键的TCF曲线相比,Ca O 键的TCF曲线下降更快,这表明连接GO和CSH的离子键主要由羟基提供氧位㊂这一结果与RDF分析结果一致㊂此外,Ca O键的TCF曲线大都在最初略有下降,然后几乎保持不变㊂这表明CSH中Ca和氧形成的离子键可以长时间连接㊂图6㊀Ca O键和H O键的时间相关函数Fig.6㊀Time correlation function of Ca O and H O bond第11期臧㊀芸等:基于分子动力学的氧化石墨烯对PVA 纤维-CSH 界面影响机理研究3805㊀三个界面中的氢键的TCF 曲线如图6(b)所示㊂相比于Ca O 键,氢键的TCF 曲线明显下降得更快,这也证明了离子键在界面连接中起到主导作用㊂界面之间化学键稳定性的差异解释了纤维与基体之间相互作用的差异㊂PVA 纤维和GO 之间没有稳定的离子键连接,导致PVA 纤维更容易被拉出㊂界面动态分析表明,三种界面结合强度依次为:CGP2>CP >CGP1,这说明如果GO 与PVA 纤维之间没有形成共价键,而只是以物理形式附着在PVA 纤维表面,不利于提升界面黏结性能,当PVA 纤维与GO 以共价键形式连接时,GO 才能发挥拥有更多极性氧位的优势,对界面的黏结性能才能起到增强作用㊂这与试验中的结果保持一致[23]㊂图7㊀不同界面的黏附能Fig.7㊀Adhesion energy of different interfaces 作用在PVA 纤维上的恢复力来自界面的相互作用,由黏附能提供[31]㊂在分子水平上,黏附能也可以用作表征界面黏结性能的参数㊂在CSH /PVA 模型中,只有存在CP (CSH /PVA)界面㊂而在CSH /GO /PVA 模型中虽然存在两个界面CSH /GO 和GO /PVA,但根据2.1节的分析,当纤维被拔出时,对纤维拉出行为产生影响的是GO /PVA 界面,所以仅计了CGP1(GO /PVA)界面的黏附能㊂在CSH /GO-PVA 模型中,GO 与PVA 纤维以共价键连接,只有界面CGP2(GO /CSH)影响到纤维被拉出行为㊂界面黏附能计算结果如图7所示,CGP1界面的黏附能仅为1979.3kcal /mol(1kJ =4.184kcal),而CP 界面的黏附能为3780.7kcal /mol,明显比界面CGP1要高出很多,这说明CSH /PVA 界面有更强的相互作用㊂CGP2界面的黏附能绝对值最大,为5515.9kcal /mol,这意味着当GO 与PVA 纤维形成共价键连接时,GO 对界面黏结性能起到增强作用㊂3㊀结㊀论1)PVA /CSH 界面主要是以钙氧离子键和氢键连接,而PVA /GO 界面仅依靠氢键连接㊂2)CGP2界面的黏结性能优于CP 界面和CGP1界面,其中CGP1界面的黏结性能最差㊂3)当PVA 纤维与GO 以共价键方式连接时,GO 发挥多极性氧位的优势,与CSH 之间形成更强的键合作用,从而加强了有机相和无机相之间的界面连接㊂当GO 以物理形式附着在PVA 纤维表面时,对界面的黏结性能起负面作用㊂参考文献[1]㊀杨宇林.纤维混凝土复合材料耐久性能研究综述[J].混凝土,2012(2):78-80+85.YANG Y L.Review on durability of complex fiber concrete[J].Concrete,2012(2):78-80+85(in Chinese).[2]㊀KANDA T,LI V C.Practical design criteria for saturated pseudo strain hardening behavior in ECC [J].Journal of Advanced ConcreteTechnology,2006,4(1):59-72.[3]㊀KONSTA-GDOUTOS M S,METAXA Z S,SHAH S P.Multi-scale mechanical and fracture characteristics and early-age strain capacity of highperformance carbon nanotube /cement nanocomposites[J].Cement and Concrete Composites,2010,32(2):110-115.[4]㊀KONSTA-GDOUTOS M S,METAXA Z S,SHAH S P.Highly dispersed carbon nanotube reinforced cement based materials[J].Cement andConcrete Research,2010,40(7):1052-1059.[5]㊀SUN G X,LIANG R,LU Z Y,et al.Mechanism of cement /carbon nanotube composites with enhanced mechanical properties achieved byinterfacial strengthening[J].Construction and Building Materials,2016,115:87-92.[6]㊀PAN Z,HE L,QIU L,et al.Mechanical properties and microstructure of a graphene oxide-cement composite [J].Cement and ConcreteComposites,2015,58:140-147.[7]㊀LV S H,MA Y J,QIU C C,et al.Effect of graphene oxide nanosheets of microstructure and mechanical properties of cement composites[J].Construction and Building Materials,2013,49:121-127.[8]㊀LU Z Y,HOU D S,MA H Y,et al.Effects of graphene oxide on the properties and microstructures of the magnesium potassium phosphate3806㊀水泥混凝土硅酸盐通报㊀㊀㊀㊀㊀㊀第42卷cement paste[J].Construction and Building Materials,2016,119:107-112.[9]㊀GEIM A K,NOVOSELOV K S.The rise of graphene[J].Nature Materials,2007,6(3):183-191.[10]㊀LU Z Y,CHEN G C,HAO W B,et al.Mechanism of UV-assisted TiO2/reduced graphene oxide composites with variable photodegradation ofmethyl orange[J].RSC Advances,2015,5(89):72916-72922.[11]㊀LONG W J,WEI J J,MA H Y,et al.Dynamic mechanical properties and microstructure of graphene oxide nanosheets reinforced cementcomposites[J].Nanomaterials,2017,7(12):407.[12]㊀何㊀威,许吉航.少层石墨烯对普通混凝土性能的影响[J].硅酸盐通报,2021,40(5):1477-1488.HE W,XU J H.Effect of few-layer graphene on properties of ordinary concrete[J].Bulletin of the Chinese Ceramic Society,2021,40(5): 1477-1488(in Chinese).[13]㊀陈㊀旭,汉光昭,裴玉胜,等.不同含量石墨烯对混凝土抗冻性能的影响[J].建筑施工,2021,43(8):1659-1663.CHEN X,HAN G Z,PEI Y S,et al.Influence of different content of graphene on frost resistance of concrete[J].Building Construction,2021, 43(8):1659-1663(in Chinese).[14]㊀徐义洪,范颖芳.氧化石墨烯分散液对混凝土抗盐冻性能的影响[J].混凝土,2022(2):1-5.XU Y H,FAN Y F.Effects of graphene oxide dispersion on the salt-frost resistance of concrete[J].Concrete,2022(2):1-5(in Chinese).[15]㊀李相国,任钊锋,徐朋辉,等.氧化石墨烯复合PVA纤维增强水泥基材料的力学性能及耐久性研究[J].硅酸盐通报,2018,37(1):245-250.LI X G,REN Z F,XU P H,et al.Research on mechanical properties and durability of graphene oxide composite PVA fiber reinforced cement-based material[J].Bulletin of the Chinese Ceramic Society,2018,37(1):245-250(in Chinese).[16]㊀JIANG W G,LI X G,LV Y,et al.Cement-based materials containing graphene oxide and polyvinyl alcohol fiber:mechanical properties,durability,and microstructure[J].Nanomaterials,2018,8(9):638.[17]㊀YAO X P,SHAMSAEI E,CHEN S J,et al.Graphene oxide-coated poly(vinyl alcohol)fibers for enhanced fiber-reinforced cementitiouscomposites[J].Composites Part B:Engineering,2019,174:107010.[18]㊀WANG P,QIAO G,ZHANG Y,et al.Molecular dynamics simulation study on interfacial shear strength between calcium-silicate-hydrate andpolymer fibers[J].Construction and Building Materials,2020,257:119557.[19]㊀PELLENQ R J M,KUSHIMA A,SHAHSAVARI R,et al.A realistic molecular model of cement hydrates[J].Proceedings of the NationalAcademy of Sciences of the United States of America,2009,106(38):16102-16107.[20]㊀MANZANO H,MOEINI S,MARINELLI F,et al.Confined water dissociation in microporous defective silicates:mechanism,dipole distribution,and impact on substrate properties[J].Journal of the American Chemical Society,2012,134(4):2208-2215.[21]㊀ALLINGTON R D,ATTWOOD D,HAMERTON I,et al.A model of the surface of oxidatively treated carbon fibre based on calculations ofadsorption interactions with small molecules[J].Composites Part A:Applied Science and Manufacturing,1998,29(9/10):1283-1290. [22]㊀JIAO S P,XU Z P.Selective gas diffusion in graphene oxides membranes:a molecular dynamics simulations study[J].ACS Applied Materials&Interfaces,2015,7(17):9052-9059.[23]㊀LU C,LU Z Y,LI Z J,et al.Effect of graphene oxide on the mechanical behavior of strain hardening cementitious composites[J].Constructionand Building Materials,2016,120:457-464.[24]㊀WANG P,QIAO G,HOU D S,et al.Functionalization enhancement interfacial bonding strength between graphene sheets and calcium silicatehydrate:insights from molecular dynamics simulation[J].Construction and Building Materials,2020,261:120500.[25]㊀SHAHRIYARI R,KHOSRAVI A,AHMADZADEH A.Nanoscale simulation of Na-Montmorillonite hydrate under basin conditions,application ofCLAYFF force field in parallel GCMC[J].Molecular Physics,2013,111(20):3156-3167.[26]㊀KUNDALWAL S,KUMAR S.Multiscale modeling of stress transfer in continuous microscale fiber reinforced composites with nano-engineeredinterphase[J].Mechanics of Materials,2016,102:117-131.[27]㊀KUNDALWAL S,MEGUID S.Multiscale modeling of regularly staggered carbon fibers embedded in nano-reinforced composites[J].EuropeanJournal of Mechanics-A Solids,2017,64:69-84.[28]㊀HOU D S,YANG Q R,JIN Z Q,et al.Enhancing interfacial bonding between epoxy and CSH using graphene oxide:an atomistic investigation[J].Applied Surface Science,2021,568:150896.[29]㊀ALLEN M P,TILDESLEY D puter simulation of liquids[M].New York:Oxford University Press,2017.[30]㊀LU Z,YU J,YAO J,et al.Experimental and molecular modeling of polyethylene fiber/cement interface strengthened by graphene oxide[J].Cement and Concrete Composites,2020(112):103676.[31]㊀LI X Y,LU Z Y,CHUAH S,et al.Effects of graphene oxide aggregates on hydration degree,sorptivity,and tensile splitting strength of cementpaste[J].Composites Part A:Applied Science and Manufacturing,2017,100:1-8.。
氧化石墨烯荧光淬灭

报告人:蔡向东
1.ABSTRACT
Traditional methods of assessing kinase acitivities Graphene&Graphene Oxide
2.EXPERIMENTAL SECTION &RESULTS AND DISCUSSION
Graphite oxide,staurosporine, forskolin,CKII, IBMX,PKA,CPY,H-89, BSA(NIUXUEQING),Tris, glycerol, DTT, EDTA, and Triton X-100,FITC-pep, FITC-Ppep, FITCkemptide Synergy Mx multimode microplate reader excitation wavelength of 480 nm, fluorescence emission was scanned from 500 to 700 nm
3Q
Hydrolyzation of Peptide by CPY.
Fluorescence responses at 520 nm emission of 10 μM FITC-labeled peptide (S-pep) digested by different concentrations of CPY for 30 min and then mixed with GO.
Fluorescence Quenching of FITC Molecules and FITC-peptide by Graphene Oxide
AFM topographic images (top) and height profiles (bottom) of GO (A) before and (B) after incubation with the 10 μM peptide substrate of CKII.
水合肼与抗坏血酸还原氧化石墨烯

水合肼与抗坏血酸还原氧化石墨烯吕亚楠1,2,夏林1,陶呈安1,朱慧1,王建方1,*1国防科技大学理学院化学与生物学系,湖南长沙,4100732湖南师范大学化学化工学院,湖南长沙,410081*Email: wangjianfang@2004年Geim等发现了石墨烯的电场效应后,石墨烯的研究发展十分迅速,展现出了诱人的发展前景[1]。
目前合成石墨烯的方法主要有物理微机械剥离法、氧化-还原法、化学气相沉积(CVD)、SiC热解法等。
氧化还原法简便易行而且可以通过改变还原剂或者添加分散剂得到水溶液中分散良好的石墨烯。
本文首先运用改进的Hummer方法[2]制备出了氧化石墨烯,然后分别用水合肼100℃回流和抗坏血酸常温N2保护下还原得到石墨烯,并利用傅立叶红外光谱(FT-IR)、X-射线衍射分析(XRD)、透射电子显微镜(TEM)进行分析。
结果表明:抗坏血酸可以将氧化石墨上大部分含氧官能团有效去除,并且可以得到分散良好的石墨烯水分散系,该方法有望用于大量制备水溶性石墨烯。
Fig. 1Photographs of aqueous dispersions of GO(A) and RGOprepared by hydrazine hydrate(B) and ascorbic acid(C)关键词:改进的Hummer法;水合肼;抗坏血酸参考文献[1] Novoselov, K. S.; Geim, A. K.; Morozov, S. V.; Jiang, D.;Zhang, Y.; Dubonos, S. V.; Grigorieva, I. V.; Firsov, A. A. Science 2004, 306, 666-669.[2] Daniela C. Marcano, Dmitry V. Kosynkin, Jacob M. Berlin, Alexander Sinitskii, Zhengzong Sun,Alexander Slesarev, Lawrence B. Alemany, Wei Lu, and James M. Tour.Nano letter.2010,4(8). 4806-4814.Reduction of Graphene Oxidevia Hydrazine Hydrate and Ascorbic AcidLv Ya'nan1,2, Xia Lin1,Tao Cheng'an1, Zhu Hui1, Wang Jianfang1,*1College of Science, National University of Defense Technology, Changsha, 410073 2College of Chemistry and Chemical Engineering , Hunan NormalUniversity,Changsha,410081In this paper, we prepared graphite oxide powder by modified Hummer method, then reduced℃ascorbic acid at room temperature it respectively via hydrazine hydrate refluxing at the 100 andunder the protection of N2. The products were characterized by FT-IR , XRD, TEM. Results indicate that ascorbic acid can remove most of the oxygen functionalities of GO, and we can obtain better RGO aqueous dispersions. This method is more promising in large scale production of water soluble graphene.。
- 1、下载文档前请自行甄别文档内容的完整性,平台不提供额外的编辑、内容补充、找答案等附加服务。
- 2、"仅部分预览"的文档,不可在线预览部分如存在完整性等问题,可反馈申请退款(可完整预览的文档不适用该条件!)。
- 3、如文档侵犯您的权益,请联系客服反馈,我们会尽快为您处理(人工客服工作时间:9:00-18:30)。
Supporting InformationExfoliation of graphite oxide in propylene carbonate and thermal reductionof the resulting graphene oxide plateletsBy Yanwu Zhu, Meryl D. Stoller, Weiwei Cai, Aruna Velamakanni, Richard D. Piner, David Chen, and Rodney S. Ruoff*1. Effect of pH values on the dispersion of GO in PCIt was found that the pH of the graphite oxide (GO) has a significant effect on its dispersion extent in propylene carbonate (PC). During the preparation of GO powder, 10% HCl solution in water (200 ml) was used to wash the GO slurry before drying in vacuum. Thus the as-made GO dispersed in water or PC has a pH of about 3 (for 1 mg/ml) (hereafter we shall label it as pH3 GO). To tune the pH of GO, pH3 GO was re-dispersed in water by magnetic stirring (no sonication) and diluted ammonium hydroxide (15% concentration) was added with a drop step till the pH reached 5, 7 or 10, respectively.Then, the GO suspensions with pH 5, pH 7 and pH 10 were filtered and dried in air; thus we obtained pH5 GO, pH7 GO and pH10 GO plate-like samples on filter membranes. The pH5 GO, pH7 GO and pH10 GO samples were subject to further drying in vacuum for two days. Following the same process described in the experimental section, 20 mg of dry pH5 GO, pH7 GO and pH10 GO were dispersed in 20 ml PC each and sonicated for 2 hours in the bath sonicator (VWR B2500A-MT). As can be seen from Fig. S1, pH10 GO has almost no dispersion in PC while pH7 GO has a small degree of dispersion degree in PC as observed by changing the color of PC to light brown. The pH5 GOshowed a similar dispersion with pH3 GO at this concentration (1 mg/ml). The highest concentrations of dispersed/exfoliated pH3 GO, pH7 GO and pH10 GO in PC were estimated as 1.3, 0.05 mg/ml and 0.01 mg/ml, respectively. As a comparison, pH3 GO dispersion has a highest concentration of about 3 mg/ml.Fig. S1 Optical images of GO with variable pH values in PC, after 2 hours of bath sonication.2. High-resolution transmission electron microscopy of the reduced graphene oxideFig. S2 Typical HRTEM image of the RG-O platelets from the 150 o C treatment. From the fringes of the folded regions, we can see that this region of the sample is composed of a stack of RG-O platelets.3. FT-IR of pure PC, GO and RG-O paperFT-IR was done to investigate the functional groups and to detect any residual PC in the RG-O paper obtained by heating graphene oxide suspension in PC at 150 o C followed by drying in vacuum at 80 o C for 2 days. Fig. S3 shows the spectra of pure PC, as-made GO and RG-O paper. All samples were made by mixing (for PC) or grounding (for GO and RG-O) with KBr. Those features at around 1044, 1610, 1708 and 3400 cm-1were observed for the GO sample, corresponding to the C-O (v(epoxy or alkoxy)), C=C (possibly due to skeletal vibrations of unoxidized graphitic domains), C=O in carboxylicacid and carbonyl moieties (v(carbonyl)), and O-H stretching mode of intercalated water, respectively.1, 2 Clearly, after thermal treatment in PC followed by drying, most of these transmission features were nearly eliminated in the spectrum of RG-O sample. Moreover, no significant PC trace was observed in the RG-O spectrum.Fig. S3 FT-IR spectra of PC, GO and RG-O.4. Comparison of the conductivity of paper-like samples composed of ‘RG-O’ from different fabrication/reduction methodsTable S1Materials/reduction Method Electrical properties Reference Heating GO (50-90 o C) in alkaline conditions Unknown 3Hydrothermal treatment of GO in supercritical water (120-180 o C) Conductive (powder resistance of ~40 Ω)4Reduction of GO by sodium borohydride Highest conductivity of 45 S/m 5 Flash reduction of GO Conductivity of ~ 1000 S/m 2 Hydrazine reduction of GO Powder conductivity of 200 S/m 6 Hydrazine reduction of KOH-modifiedgraphene oxideConductivity of 690 S/m 7Hydrazine reduction of GO in DMF/water mixture (HRG) Air drying 1700 S/mAnnealing at 150 o C 16000 S/m8Hydrazine reduction of GO in ammoniasolutionConductivity of ~ 7200 S/m 9Pre-reduction of GO by NaBH4 ---sulphonation --- Post reduction with hydrazineConductivity of 1250 S/m 10Pre-redcution of GO by NaBH4 --- Heating inH2SO4 --- Post annealing at 1100 o C in Ar/H2Conductivity of 20200 S/m 11Rapid heating of GO up to 1050 o C Powder conductivity of 1000-2300 S/m 12Reduced graphene oxide in PC at 200 o C~ 1800 S/mAnnealing at 250 o C ~2640 S/mPresent workReduced graphene oxide in PC at 150 o C~ 2100 S/mAnnealing at 250 o C ~ 5230 S/mPresent work5. Calculation of capacitance from CV and galvanostatic curvesCapacitance values were calculated from the CV curves by dividing the current by the voltage scan rate, C = I/(dV/dt). Specific capacitance reported is the capacitance for the mass of RG-O in one electrode (specific capacitance = capacitance of single electrode / weight RG-O material of a single electrode), as per the normal convention. Capacitance as determined from galvanostatic charge/discharge was measured using C = I/(dV/dt) with dV/dt calculated from the slope of the discharge curve.6. References:1. Guo, H.-L.; Wang, X.-F.; Qian, Q.-Y.; Wang, F.-B.; Xia, X.-H. A Green Approach to the Synthesis of Graphene Nanosheets. Acs Nano 2009,3, 2653–2659.2. Cote, L. J.; Cruz-Silva, R.; Huang, J. Flash Reduction and Patterning of Graphite Oxide and Its Polymer Composite. J. Am. Chem. Soc. 2009,131, 11027-11032.3. Fan, X. B.; Peng, W. C.; Li, Y.; Li, X. Y.; Wang, S. L.; Zhang, G. L.; Zhang, F.B. Deoxygenation of Exfoliated Graphite Oxide under Alkaline Conditions: A Green Route to Graphene Preparation. Adv. Mater. 2008,20, 4490-4493.4. Zhou, Y.; Bao, Q.; Tang, L. A. L.; Zhong, Y.; Loh, K. P. Hydrothermal Dehydration for the Green Reduction of Exfoliated Graphene Oxide to Graphene and Demonstration of Tunable Optical Limiting Properties. Chem. Mater. 2009,21, 2950-2956.5. Shin, H.-J.; Kim, K. K.; Benayad, A.; Yoon, S.-M.; Park, H. K.; Jung, I.-S.; Jin, M. H.; Jeong, H.-K.; Kim, J. M.; Choi, J.-Y.; Lee, Y. H. Efficient Reduction of Graphite Oxide by Sodium Borohydride and Its Effect on Electrical Conductance. Adv. Func. Mater. 2009,19, 1987-1992.6. Stankovich, S.; Dikin, D. A.; Piner, R. D.; Kohlhaas, K. A.; Kleinhammes, A.; Jia, Y.; Wu, Y.; Nguyen, S. T.; Ruoff, R. S. Synthesis of Graphene-based Nanosheets via Chemical Reduction of Exfoliated Graphite Oxide. Carbon 2007,45, 1558-1565.7. Park, S.; An, J. H.; Piner, R. D.; Jung, I.; Yang, D. X.; Velamakanni, A.; Nguyen, S. T.; Ruoff, R. S. Aqueous Suspension and Characterization of Chemically Modified Graphene Sheets. Chem. Mater. 2008,20, 6592-6594.8. Park, S.; An, J. H.; Jung, I. W.; Piner, R. D.; An, S. J.; Li, X. S.; Velamakanni, A.; Ruoff, R. S. Colloidal Suspensions of Highly Reduced Graphene Oxide in a Wide Variety of Organic Solvents. Nano Lett. 2009,9, 1593-1597.9. Li, D.; Muller, M. B.; Gilje, S.; Kaner, R. B.; Wallace, G. G. Processable Aqueous Dispersions of Graphene Nanosheets. Nat. Nanotech. 2008,3, 101-105.10. Si, Y.; Samulski, E. T. Synthesis of Water Soluble Graphene. Nano Lett. 2008,8, 1679-1682.11. Gao, W.; Alemany, L. B.; Ci, L.; Ajayan, P. M. New Insights into the Structure and Reduction of Graphite Oxide. Nat. Chem. 2009,1, 403-408.12. Schniepp, H. C.; Li, J. L.; McAllister, M. J.; Sai, H.; Herrera-Alonso, M.; Adamson, D. H.; Prud'homme, R. K.; Car, R.; Saville, D. A.; Aksay, I. A. Functionalized Single Graphene Sheets Derived from Splitting Graphite Oxide. J. Phys. Chem. B 2006, 110, 8535-8539.。