CRYSTALLINE AND ELECTRONIC STRUCTURE OF GOLD NANOCLUSTERS
聚合物基复合固态电解质填料研究进展

㊀第56卷第3期郑州大学学报(理学版)Vol.56No.3㊀2024年5月J.Zhengzhou Univ.(Nat.Sci.Ed.)May 2024收稿日期:2023-08-25基金项目:河南省重点研发与推广专项(232102240007)㊂第一作者:唐菲(2000 ),女,硕士研究生,主要从事固态电解质研究,E-mail:1765701108@㊂通信作者:王柳(1989 ),女,讲师,主要从事固态电池及电解质研究,E-mail:wangliu@㊂聚合物基复合固态电解质填料研究进展唐㊀菲,㊀王㊀柳(郑州大学河南先进技术研究院㊀河南郑州450003)摘要:聚合物基固态电解质具有优异的稳定性㊁加工性和低成本,是实现全固态锂电池的理想电解质,然而低离子电导率严重阻碍了其应用㊂加入无机填料,构筑聚合物基复合固态电解质是提升离子电导率的有效策略㊂首先,讨论了聚合物电解质的离子传导机理及复合固态电解质组分间的协同作用㊂其次,从机械强度㊁电化学稳定窗口㊁离子电导率㊁锂离子迁移数四个方面阐述了填料的作用,并以惰性填料㊁活性填料㊁功能性填料为分类对近些年填料的研究进展进行了系统介绍,阐释了其物理化学性质对电解质性能的调控机制㊂最后,在总结现有研究结果的基础上,针对关键问题对未来的研究方向进行了展望㊂关键词:固态锂电池;复合聚合物电解质;填料;固态电解质中图分类号:TM912文献标志码:A文章编号:1671-6841(2024)03-0033-08DOI :10.13705/j.issn.1671-6841.2023195Research Progress of Fillers for Polymer-based CompositeSolid-state ElectrolytesTANG Fei,WANG Liu(Henan Institute of Advanced Technology ,Zhengzhou University ,Zhengzhou 450003,China )Abstract :Polymer-based solid-state electrolytes were ideal electrolytes for realizing all-solid-state lithiumbatteries due to their excellent stability,processability,and low cost.However,the low ionic conductivi-ty seriously hindered their application.Adding inorganic fillers and constructing polymer-based composite solid-state electrolytes was an effective strategy to improve the ionic conductivity.Firstly,the ionic con-duction mechanism of polymer electrolytes and the synergistic effect of the components in composite solid-state electrolytes were discussed.Secondly,the functions of fillers in terms of mechanical strength,elec-trochemical stabilization window,ionic conductivity,and lithium-ion transfer number were described.Furthermore,the research progress of fillers in recent years was introduced by categorizing them into inert fillers,active fillers and functional fillers,and the regulating mechanism of their physicochemical proper-ties on the electrolyte performance was explained.Finally,on the basis of summarizing the results of the existing research,the future research direction was discussed with respect to the key issues.Key words :solid-state lithium battery;composite polymer electrolyte;filler;solid-state electrolyte0㊀引言全球化石燃油消耗量日益增加,由此造成的不良气候变化和环境破坏是对人类生活的严峻挑战[1]㊂鉴于此,迫切需要一种清洁高效的设备用于可再生能源的储存与转换[2]㊂在各种电化学储能装置中,锂离子电池自20世纪90年代被索尼公司商业化以来,得到了广泛的研究和应用[3]㊂经过30年的发展,基于石墨阳极和过渡金属氧化物阴极的锂离子电池已经郑州大学学报(理学版)第56卷接近其能量密度极限(约300Wh/kg)[4],难以提供足够驱使电动汽车快速发展的能量密度㊂近年来,由于金属锂负极具有超高理论比容量和极低氧化还原电位,以其为负极的金属锂电池重新成为研究热点㊂尤其是搭载高容量正极的锂硫电池和锂空气电池,被认为是最有希望突破能量密度限制的下一代储能装置[5]㊂目前商业化的电池均基于液态电解质,由于其具有高离子电导率和良好的电极表面润湿性[6],电池表现出优异的电化学性能㊂然而,基于高挥发性和可燃性有机溶剂的液态电解质离子选择性低㊁稳定性差,且循环时阳极侧不断生长的枝晶会刺穿隔膜导致电池短路失效[7],使用液态锂电池时存在安全隐患㊂因此,设计抑制枝晶生长和高安全性的新型电解质有望突破高能量密度锂电池的瓶颈㊂固态电解质具有优异的机械性能,能有效抑制锂枝晶的生长㊂此外,与液态电解质相比,其具有不易燃㊁无泄露的优点,提高了电池的安全性㊂全固态锂电池的应用进一步提高了能量/功率密度,降低了对封装状态和监测电路的要求[8]㊂更值得一提的是,固态电解质能够防止锂硫电池中可溶性多硫化物的穿梭效应[9],并保护锂金属阳极免受锂硫电池中大气环境的腐蚀[10]㊂由于这些优点,近年来对固态电解质的研究呈快速增长的趋势㊂目前,固态电解质可分为以下三类:无机固态电解质㊁聚合物固态电解质㊁复合聚合物电解质㊂无机固态电解质中最具有代表性的是氧化物和硫化物电解质,其具有极高的离子电导率(10-4~10-2S/cm),能够媲美液态电解质㊂但硫化物电解质存在严重的分解现象,氧化物电解质具有很大的界面电阻,这阻碍了无机固态电解质的实际应用㊂聚合物固态电解质如聚氧化乙烯(PEO)电解质,具有优异的柔韧性和可加工性,但存在室温离子电导率低㊁机械强度差㊁热稳定性差等问题,严重限制了其应用㊂聚合物电解质的结构改性已经取得重大进展,但在提升离子电导率方面仍面临巨大挑战[5]㊂近年来,研究者们将聚合物㊁锂盐和填料混合形成复合聚合物电解质,它不仅具有聚合物电解质优异的柔韧性和可加工性,同时由于填料的加入,实现了离子电导率的提高,弥补了聚合物电解质的缺陷[11]㊂复合聚合物电解质中的填料通过降低聚合物结晶度㊁促进锂盐解离和构建新的离子传导通道等方式来达到高离子电导率㊂本文首先介绍了复合聚合物电解质的离子传导机理,然后系统地介绍了不同种类的填料对电解质性能的影响及其作用机理,最后展望了聚合物基复合固态电解质的发展前景㊂1㊀复合聚合物电解质离子传导机理聚合物电解质具有多相结构,其离子传导非常复杂㊂首先是不同相共存,如非晶相和PEO与锂离子的各种晶体配合物,为离子传导提供了不同途径;其次是相的分布和结构复杂㊂在以往实验和理论研究中已经提出多种运输机制,如锂离子与醚氧(EO)之间形成弱配位壳的阳离子跳跃,以及PEO熔融相中自由离子沿渗透通道运输㊂在不同的控制因素中,聚合物主链的链段运动被认为是影响锂离子和阴离子迁移的关键因素[12]㊂在聚合物电解质中,锂离子能和聚合物链上的极性基团(如醚氧键)配位[13]㊂PEO聚合物固态电解质中锂离子传导机制如图1所示㊂在电场作用下,锂离子通过配位键的断裂和形成,沿分子链或跨分子链从原配位位点跳跃到新的配位位点,从而实现锂离子的传导㊂图1㊀PEO聚合物固态电解质中锂离子传导机制[12] Figure1㊀Mechanism of lithium-ion transport in PEOsolid-state electrolyte大多数聚合物电解质结晶度高,室温下其晶态和非晶态区域共存(主要以晶态形式存在)[14]㊂实际上,非晶态区域中聚合物链段运动活跃,更利于锂离子的传导,而晶态区域中链段运动受到限制,锂离子传导十分缓慢㊂因此,在室温下聚合物电解质的离子电导率通常很低㊂而加热时无定形聚合物表现出从 玻璃状 到 橡胶状 的转变,冷却时则反之㊂在玻璃状态下,它们是刚性聚合物链;在橡胶状态下,聚合物链变得更柔韧,链段运动被激活㊂这种转变发生在玻璃化转变温度(T g),这是聚合物电解质的核心性质之一㊂聚合物链的迁移率是离子传导的关键㊂在T g以下,聚合物链在很大程度上是刚性和不移动的,无定形聚合物具有接近零的离子电导率㊂因此,聚合物电解质在T g附近或以下的导电性非常差;在T g以上,局部聚合物链运动实际上是液体状且快速的㊂但是,由于受到高T g和结晶度的限制,43㊀第3期唐㊀菲,等:聚合物基复合固态电解质填料研究进展聚合物电解质的离子电导率非常低㊂在聚合物电解质中引入无机填料可以得到聚合物基复合电解质,复合电解质已被证明更有希望推动固态锂电池的发展[15]㊂加入无机填料之后,复合聚合物电解质中离子迁移速率明显加快,这得益于填料的以下功能:1)与聚合物相互作用,降低聚合物的T g 和结晶度㊂2)与锂盐发生路易斯酸碱或其他相互作用,从而促进锂盐解离,使自由锂离子数量增加㊂3)通过特殊官能团与锂离子和聚合物配合,减弱锂离子与聚合物之间的相互作用,从而降低聚合物链段运动中锂离子跳跃的能量势垒㊂4)构建新的锂离子迁移通道(通过填料-聚合物界面相或渗透活性填料)㊂2㊀填料的作用机械强度㊁电化学稳定窗口㊁离子电导率㊁锂离子迁移数是衡量固态电解质电化学性能的重要指标㊂在聚合物电解质中加入无机填料可以优化这些性能,但优化程度与填料参数,如填料类型㊁填料尺寸㊁填料含量等有关[11]㊂下文将详细讨论聚合物基复合固态电解质电化学性能的增强机理㊂2.1㊀机械强度机械强度是大批量电池生产中最重要的参数㊂聚合物基复合电解质应具备使电池有望大规模制造的机械性能,以减小循环时的体积变化并抑制锂枝晶生长㊂理论上,剪切模量>6GPa的复合电解质(无孔隙和晶界)能够抑制锂枝晶生长㊂聚合物电解质剪切模量较低(通常<0.1GPa[16]),因此难以阻断锂枝晶㊂但无机电解质的剪切模量能够达到数十GPa(LLZO约为60GPa[17])㊂通常情况下,加入无机填料增加了聚合物基的抗拉强度,但降低了断裂伸长率㊂当电解质中陶瓷填料含量过多时,复合聚合物电解质的柔韧性和黏附性急速下降,黏附性差将破坏电极与电解质之间的紧密接触,致使充放电过程中界面阻抗增大[18]㊂选择一种黏性㊁自愈性和成膜性良好的聚合物有望实现具有高机械稳定性的复合聚合物电解质㊂以下方法可作为提高机械性能的策略:在复合聚合物电解质中添加二维/三维材料;设计交联结构或加入刚性基团;采用三维结构设计复合聚合物电解质㊂2.2㊀电化学稳定窗口电化学稳定窗口是指电解质不发生氧化还原分解的稳定电压范围㊂具有宽电化学稳定窗口的电解质能保证电池在高电压下稳定运行,这对实现高能量密度锂电池具有重要意义㊂对于大多数聚合物电解质,由于聚合物基体在阳极分解,其高压稳定电位在室温下不超过4.2V㊂例如,由于末端羟基较活泼,最常见的PEO聚合物电解质在超过3.8V时开始发生缓慢氧化[19]㊂而用无机填料改性的PEO基复合电解质甚至可承受高达5V的电压,这可能是由于无机填料表面的某些基团与PEO中的活性基团之间形成了新的化学键,从而抑制其氧化[5]㊂一般来说,在聚合物电解质中加入无机填料可以提高其高压稳定电位,可能的原因如下:1)无机填料表面的某些基团与聚合物基体的活性基团或阴离子形成化学键,防止其氧化㊂2)无机填料表面的酸性基团通过路易斯酸碱相互作用固定阴离子,减少其在电极上的积累㊂3)无机填料本身的电化学稳定窗口非常宽㊂例如,LLZO 和LLTO具有超过5V的高压稳定电位[20]㊂4)无机填料可以去除聚合物基体中的部分杂质㊂由此可知,无机填料与聚合物基体之间的相互作用能有效提高聚合物电解质的电化学稳定性㊂故可通过以下策略来提高聚合物电解质的电化学稳定性:添加比表面积大的填料与聚合物基体形成更多的界面区域;表面具有丰富的酸性基团或酸性位点的填料与聚合物基体以多种形式相互作用;加入高热力学㊁动力学稳定性的组分作为填料㊂2.3㊀离子电导率在聚合物电解质中加入无机填料是提高其离子电导率的一种简便而有效的方法,添加无机填料能够将聚合物电解质的室温离子电导率提高1~3个数量级[21]㊂Hu等[22]通过选择性同位素标记和高分辨率固态Li核磁共振谱进行了深入研究,研究结果表明,锂离子可以在聚合物基体㊁活性填料和填料-聚合物界面区域迁移,且锂离子的迁移速率受聚合物基复合电解质组成和填料含量的影响㊂此外,不同填料对聚合物电解质离子电导率的增强机制也有显著差异㊂其中,惰性填料提高离子电导率的主要原因如下:1)降低聚合物结晶度,通过促进聚合物链段运动来加速锂离子迁移㊂2)填料-阴离子存在静电相互作用,能够削弱阴离子对锂离子的束缚[22]㊂3)填料-聚合物之间存在路易斯酸碱相互作用,促进填料-聚合物界面上形成新的锂离子传输路径,有效提高锂离子传导速率[23]㊂许多研究结果表明,无机填料与聚合物基体之间的界面区域具有较高的离子电导率,能显著提高聚合物基复合电解质的离子电导率㊂例如,0ħ时P(EO)16LiTFSI与Al2O3界面上离子电导率高达10-3S/cm[24],30ħ时PAN/LiClO4与LLTO纳米线53郑州大学学报(理学版)第56卷界面上离子电导率为1.26ˑ10-2S/cm[20]㊂相较于惰性填料,活性填料为锂离子传导提供了新路径,即填料内部连续的锂离子迁移通路,故其能够作为很好的提高离子电导率的材料㊂具有特殊结构的功能性填料,如二维层状材料,COF㊁MOF等多孔材料,其加入聚合物基体中还能弱化锂离子和聚合物链的相互作用,使锂离子能够在填料的自身空间如二维材料层间,形成新的相互作用,创造新的离子迁移通道,进而大幅提高离子传导速率㊂综上所述,要实现聚合物基复合电解质的高离子电导率,降低聚合物结晶度㊁促进锂盐解离㊁建立丰富的离子传导通道等方法都具有可行性㊂2.4㊀锂离子迁移数锂离子迁移数反映了锂离子迁移在总离子电导率中的贡献率㊂大多数电解质都是自由锂离子和自由阴离子共存,其离子电导率由锂离子和阴离子传导共同参与㊂电池循环时,锂离子和阴离子都能迁移,但阴离子迁移方向与锂离子相反㊂同时,由于阴离子与锂离子的静电相互作用,导致在阳极到阴极的方向上形成很大的锂离子浓度梯度,这阻碍了锂离子的迁移并导致死锂沉积和枝晶生长㊂另一类单离子导体电解质虽然锂离子迁移数很高,能实现锂的均匀生长,有效防止生成锂枝晶,但存在严重的界面问题,影响了电池性能㊂锂离子与聚合物极性基团紧密配位,聚合物电解质中锂离子的流动性比阴离子小㊂因此,聚合物电解质的锂离子迁移数通常很低㊂在聚合物电解质中加入无机填料可以一定程度地提高聚合物电解质的锂离子迁移数,无机填料增加锂离子迁移数可能归因于以下两个方面:1)无机填料的酸性基团或位点通过路易斯酸碱相互作用固定阴离子,限制了阴离子运动㊂2)无机填料的酸性基团或位点与聚合物极性基团相互作用,削弱了锂离子与极性基团之间的配位,促进锂离子迁移㊂3㊀填料的分类根据填料自身能否传导锂离子,可以将填料分为惰性填料㊁活性填料[25]和具有独特结构的功能性填料[26],这些填料能够提高离子电导率㊁锂离子迁移数㊁电化学稳定窗口和力学性能㊂不同填料在改性聚合物电解质时侧重于不同方面,接下来详细介绍三种填料的异同点㊂3.1㊀惰性填料惰性填料是指本身不能传导锂离子的材料,如典型的陶瓷氧化物(Al2O3㊁TiO2和SiO2等)[22]㊂惰性填料表面含有丰富的官能团,能与聚合物基体形成路易斯酸碱相互作用,从而阻碍聚合物结晶,增加电解质中的非晶区域,促进聚合物链段的运动[27],同时还能优化聚合物电化学稳定性;能与锂盐的阴阳离子形成相互作用(如氢键相互作用㊁偶极-偶极相互作用等)来促进锂盐解离,还能通过静电相互作用固定阴离子,提高锂离子的活动性,进而提高电解质的离子电导率和锂离子迁移数㊂惰性填料在聚合物电解质中的相互作用如图2所示[28-30]㊂图2㊀惰性填料在聚合物电解质中的相互作用Figure2㊀Interaction of inert fillers in polymer electrolytes63㊀第3期唐㊀菲,等:聚合物基复合固态电解质填料研究进展㊀㊀增加填料表面缺陷能调节聚合物/锂盐的相互作用㊁锂离子局部环境及锂离子迁移能垒[28]㊂将Gd 0.1Ce 0.9O 1.95(GDC)和钙钛矿La 0.8Sr 0.2Ga 0.8Mg 0.22O 2.55(LSGM)两种商用锂离子绝缘氧化物作为无机填料引入PEO 中,30ħ时聚合物基复合电解质的离子电导率提升至10-4S /cm㊂增强原因主要是这两种材料体内和颗粒表面的氧空位(路易斯酸位)㊂如图2(c)所示,为了研究氧空位对填料的影响,进行了密度泛函理论和高分辨率固态Li 核磁共振谱表征,证明富含氧空位的填料能够有效地吸附TFSI -,同时削弱PEO 中配位氧对锂离子的固定作用,极大地提高锂离子的自由度㊂可见,无机填料对聚合物电解质性能影响很大㊂图2(a )中粉煤灰[29]和图2(b)中Si 3N 4[30]具有相似的结果㊂综上所述,惰性填料通常能有效降低聚合物链的结晶程度,从而促进聚合物链段的运动,但离子电导率也与可移动锂离子的数量密切相关㊂填料表面丰富的路易斯酸作用位点能与锂盐的阴离子发生极强的相互作用,促使锂盐解离,从而有效提高聚合物基复合电解质的离子电导率㊂在未来的研究中可以设计具有丰富路易斯酸位点(如氧空位㊁羟基官能团等)的惰性填料㊂3.2㊀活性填料活性填料即无机离子导体,不仅具有惰性填料的一般功能,还能通过材料体内附加的锂离子迁移途径参与离子传导过程,从而进一步提高离子传导速率[31]㊂通常,活性填料主要包括氧化物基钙钛矿㊁NASICON 型[32]或石榴石型结构以及硫化物㊂钙钛矿型材料(Li 3x La 2/3-x TiO 3)在室温下的离子电导率高达10-3S /cm,且具有优异的高压稳定性,是目前最受欢迎的活性填料之一㊂活性填料在聚合物电解质中的作用如图3所示㊂图3㊀活性填料在聚合物电解质中的作用Figure 3㊀The role of active fillers in polymer electrolytes㊀㊀Lv 等[31]采用刚性亲水的蛭石(Vr)纳米片构建出规整的二维层间通道,然后在层间限域空间中原位形成有序排布和无明显结构缺陷的大尺寸Li 0.33La 0.557TiO 3(LLTO)晶体(图3(a)),锂离子沿着c 轴方向高速移动,在层间得到8.22ˑ10-5S /cm 的离子电导率㊂NASICON 型总体结构为LiA 2(PO 4)3(A =Ti㊁Ge㊁Zr 等),NASICON 型填料的优点主要包括优异的水和空气稳定性,相对Li /Li +有高达5V 的电化学稳定电位,但与金属锂接触时电池容易因界面反应而失效㊂Ye 等[33]制备了一种具有界面稳定性的三明治多层结构LPSCl-LGPS-LPSCl 电解质(图3(b)),其能够在不稳定的电解质层中发生趋好的局部分解,并获得优异的电化学稳定性㊂以锂金属为负极,LiNi 0.8Mn 0.1Co 0.1O 2(NCM811)为正极组装的全电池甚至在20C 倍率下仍能表现出优异的循环性能㊂石榴石型Li 7La 3Zr 2O 12(LLZO)电解质的离子电导率高(10-4~10-3S /cm ),电压窗口宽(vs.Li /Li +>5V)㊂但LLZO 与空气中的二氧化碳和水易发生反应,其表面会形成离子绝缘的Li 2CO 3层,使界面阻抗增大㊂Yang 等[25]提出一种通过表面锂供体反应得到新型陶瓷材料并与正极内部界面适配的策略㊂LLZO 近表面层及其表面的Li 2CO 3惰性层充当锂供体,作为锂源,与选定的金属氧化物反应后,得到稳定功能衍生层包覆的LLZO㊂制得的Li 6.75La 3Zr 1.75Ta 0.25O 12(LLZTO )包覆LiCoO 2(LLZTO@LCO )的离子传输性能和空气稳定性十分优异73郑州大学学报(理学版)第56卷(图3(c))㊂相比氧化物基活性填料,硫化物活性填料通常有高离子电导率和低晶界电阻㊂由于活性填料能提供更多的锂离子传输途径,离子电导率不会完全受限于聚合物的链段运动㊂然而,许多活性填料在使用锂金属阳极时存在化学不稳定性以及空气不稳定性的问题㊂通过掺杂㊁包覆或表面改性等方法可以提高活性填料的性能㊂3.3㊀功能性填料近年来出现的一些功能性填料,如COF [34]㊁MOF [21]㊁二维材料(GO㊁黏土片)等,这种填料通常具有特殊的结构和丰富的官能团,可以同时改善聚合物基复合电解质的电化学和力学性能,从而使电解质更具实际应用的潜力㊂功能性填料在聚合物电解质中的相互作用如图4所示㊂图4㊀功能性填料在聚合物电解质中的相互作用Figure 4㊀Interaction of functional fillers inpolymer electrolytesMOF 是无机金属中心(金属离子或金属团簇)与桥接的有机配体通过自组装连接而形成的具有周期网状结构的新型晶体多孔材料㊂MOF 具有可调节的多孔结构,作为填料能限制阴离子移动,促进锂离子迁移㊂同时,丰富的官能团可以与锂盐和聚合物相互作用,促进锂盐解离,锚定阴离子,削弱锂离子与聚合物的亲和力㊂相比传统固态电解质材料,MOF 固态电解质展现出高离子电导率㊁高电化学稳定性和高环境适应性㊂Wang 等[21]定向制备了具有优异电子和离子传导可调性的MOF 材料,同时将其用作光辅助锂空气电池的固态电解质和正极,有效避免了采用两种不同电子和离子导体而引起的电子转移和锂离子传导不连续的问题㊂同时,引入光照极大地降低了传统固态电池充电过程中的反应能垒,进而降低电池充电电位,优化电池能量转换效率和延长循环寿命,构筑了高安全性㊁长寿命的光辅助固态锂空气电池㊂COF 也具有高度可调的分子结构和理想有序的离子迁移通道㊂Guo 等[34]首次设计并合成了具有整体定向通道结构的柔性耐高压共价有机框架复合电解质薄膜(图4(b))㊂通过合理地引入亲锂基团和电化学稳定的喹啉基芳香环链,制备出带隙超低的COF,其HOMO 值在真空下为-6.2eV,氧化稳定性最高达5.6V㊂获得的柔性共价有机框架复合电解质薄膜显示出沿(001)面的定向排列,在60ħ时具有1.5ˑ10-4S /cm 的高离子电导率以及优异的机械强度,且使匹配富镍正极(NMC811)的固态锂金属电池成为可能㊂由此可见,创造一种新的可供锂离子高速传输的分子通道是功能性填料的重要特征㊂2021年,Yang 等[23]利用Cu 2+与纳米纤维素形成配位作用,扩大聚合物链间距从而形成分子通道,并使纤维素变为无定形结构(图4(a)),使锂离子快速地插入和迁移㊂除了具有高离子电导率(沿分子链在室温下为1.5ˑ10-3S /cm),Cu 2+配位的纳米纤维素离子导体同时具有高锂离子迁移数(0.78)以及宽电化学稳定窗口(0~4.5V)㊂综上所述,与惰性填料和活性填料不同,功能性填料通常具有丰富的官能团和可调节的分子结构㊂这些基团能够促进与聚合物和锂盐的相互作用,特别是可以极大地锚定阴离子,减弱锂离子与聚合物之间的亲和力,加速锂离子的迁移,同时实现更好的填料-聚合物界面相容性㊂功能性填料的结构特征,如分子孔和二维结构,为有效定制复合聚合物电解质中的相互作用和离子传输途径提供了参考㊂4㊀小结与展望与无机固态电解质和聚合物电解质相比,含有填料作为增强成分的聚合物基复合电解质有望同时实现高离子电导率㊁优异的力学性能以及与电极的紧密界面接触㊂本文综述了不同类型填料的特点及其对聚合物基复合电解质性能的影响机理,这些填料通过抑制聚合物链的结晶度㊁促进锂盐的解离和固定阴离子等方式来实现优异的电化学性能㊂此外,在填料内部和填料-聚合物界面处可以构建新83。
berry phases in electronic structure theory pdf
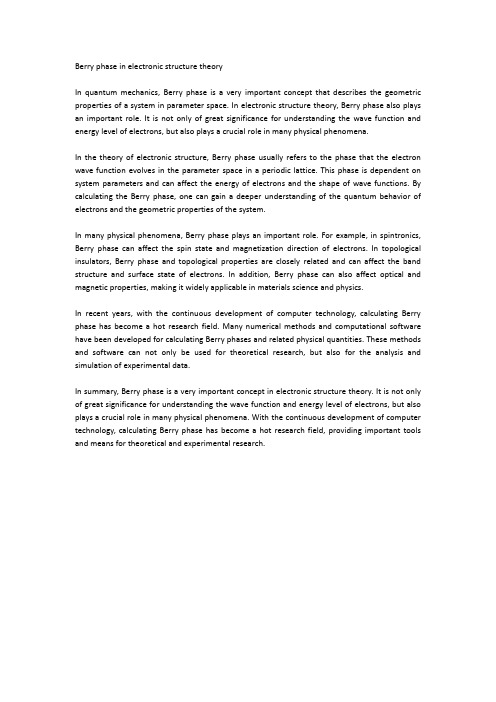
Berry phase in electronic structure theoryIn quantum mechanics,Berry phase is a very important concept that describes the geometric properties of a system in parameter space.In electronic structure theory,Berry phase also plays an important role.It is not only of great significance for understanding the wave function and energy level of electrons,but also plays a crucial role in many physical phenomena.In the theory of electronic structure,Berry phase usually refers to the phase that the electron wave function evolves in the parameter space in a periodic lattice.This phase is dependent on system parameters and can affect the energy of electrons and the shape of wave functions.By calculating the Berry phase,one can gain a deeper understanding of the quantum behavior of electrons and the geometric properties of the system.In many physical phenomena,Berry phase plays an important role.For example,in spintronics, Berry phase can affect the spin state and magnetization direction of electrons.In topological insulators,Berry phase and topological properties are closely related and can affect the band structure and surface state of electrons.In addition,Berry phase can also affect optical and magnetic properties,making it widely applicable in materials science and physics.In recent years,with the continuous development of computer technology,calculating Berry phase has become a hot research field.Many numerical methods and computational software have been developed for calculating Berry phases and related physical quantities.These methods and software can not only be used for theoretical research,but also for the analysis and simulation of experimental data.In summary,Berry phase is a very important concept in electronic structure theory.It is not only of great significance for understanding the wave function and energy level of electrons,but also plays a crucial role in many physical phenomena.With the continuous development of computer technology,calculating Berry phase has become a hot research field,providing important tools and means for theoretical and experimental research.。
材料科学基础英文词汇(最新整理)
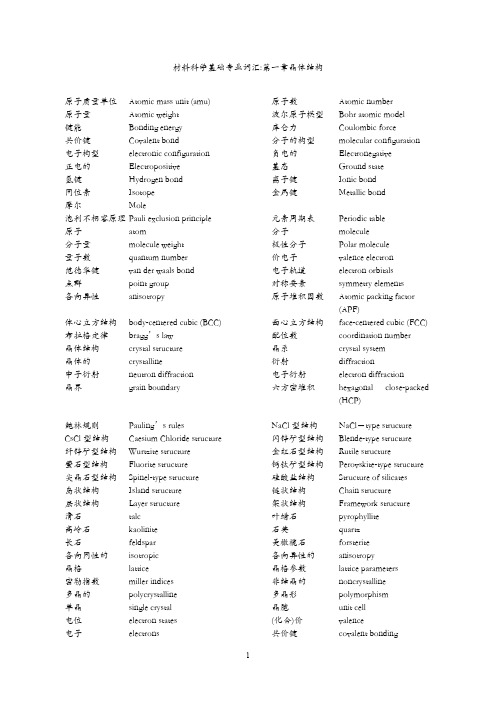
材料科学基础专业词汇:第一章晶体结构原子质量单位Atomic mass unit (amu) 原子数Atomic number原子量Atomic weight 波尔原子模型Bohr atomic model键能Bonding energy 库仑力Coulombic force共价键Covalent bond 分子的构型molecular configuration 电子构型electronic configuration 负电的Electronegative正电的Electropositive 基态Ground state氢键Hydrogen bond 离子键Ionic bond同位素Isotope 金属键Metallic bond摩尔Mole泡利不相容原理 Pauli exclusion principle 元素周期表Periodic table原子atom 分子molecule分子量molecule weight 极性分子Polar molecule量子数quantum number 价电子valence electron范德华键van der waals bond 电子轨道electron orbitals点群point group 对称要素symmetry elements各向异性anisotropy 原子堆积因数Atomic packing factor(APF)体心立方结构body-centered cubic (BCC) 面心立方结构face-centered cubic (FCC) 布拉格定律bragg’s law 配位数coordination number晶体结构crystal structure 晶系crystal system晶体的crystalline 衍射diffraction中子衍射neutron diffraction 电子衍射electron diffraction晶界grain boundary 六方密堆积hexagonal close-packed(HCP)鲍林规则Pauling’s rules NaCl型结构NaCl-type structure CsCl型结构Caesium Chloride structure 闪锌矿型结构Blende-type structure纤锌矿型结构Wurtzite structure 金红石型结构Rutile structure萤石型结构Fluorite structure 钙钛矿型结构Perovskite-type structure 尖晶石型结构Spinel-type structure 硅酸盐结构Structure of silicates岛状结构Island structure 链状结构Chain structure层状结构Layer structure 架状结构Framework structure滑石talc 叶蜡石pyrophyllite高岭石kaolinite 石英quartz长石feldspar 美橄榄石forsterite各向同性的isotropic 各向异性的anisotropy晶格lattice 晶格参数lattice parameters密勒指数miller indices 非结晶的noncrystalline多晶的polycrystalline 多晶形polymorphism单晶single crystal 晶胞unit cell电位electron states (化合)价valence电子electrons 共价键covalent bonding金属键metallic bonding 离子键Ionic bonding极性分子polar molecules 原子面密度atomic planar density衍射角diffraction angle 合金alloy粒度,晶粒大小grain size 显微结构microstructure显微照相photomicrograph 扫描电子显微镜scanning electronmicroscope (SEM)重量百分数weight percent 透射电子显微镜 transmission electronmicroscope (TEM)四方的tetragonal 单斜的monoclinic配位数coordination number材料科学基础专业词汇:第二章晶体结构缺陷缺陷defect, imperfection 点缺陷point defect线缺陷line defect, dislocation 面缺陷interface defect体缺陷volume defect 位错排列dislocation arrangement位错线dislocation line 刃位错edge dislocation螺位错screw dislocation 混合位错mixed dislocation晶界grain boundaries 大角度晶界high-angle grainboundaries 小角度晶界tilt boundary, 孪晶界twin boundaries位错阵列dislocation array 位错气团dislocation atmosphere位错轴dislocation axis 位错胞dislocation cell位错爬移dislocation climb 位错聚结dislocation coalescence位错滑移dislocation slip 位错核心能量dislocation core energy位错裂纹dislocation crack 位错阻尼dislocation damping位错密度dislocation density 原子错位substitution of a wrongatom间隙原子interstitial atom 晶格空位vacant lattice sites间隙位置interstitial sites 杂质impurities弗伦克尔缺陷Frenkel disorder 肖脱基缺陷Schottky disorder主晶相the host lattice 错位原子misplaced atoms缔合中心Associated Centers. 自由电子Free Electrons电子空穴Electron Holes 伯格斯矢量Burgers克罗各-明克符号K roger Vink notation 中性原子neutral atom材料科学基础专业词汇:第二章晶体结构缺陷-固溶体固溶体solid solution 固溶度solid solubility化合物compound 间隙固溶体interstitial solid solution置换固溶体substitutional solid solution 金属间化合物intermetallics不混溶固溶体immiscible solid solution 转熔型固溶体peritectic solid solution有序固溶体ordered solid solution 无序固溶体disordered solid solution 固溶强化solid solution strengthening 取代型固溶体Substitutional solidsolutions过饱和固溶体supersaturated solid solution 非化学计量化合物Nonstoichiometric compound材料科学基础专业词汇:第三章熔体结构熔体结构structure of melt 过冷液体supercooling melt玻璃态vitreous state 软化温度softening temperature粘度viscosity 表面张力Surface tension介稳态过渡相metastable phase 组织constitution淬火quenching 退火的softened玻璃分相phase separation in glasses 体积收缩volume shrinkage材料科学基础专业词汇:第四章固体的表面与界面表面surface 界面interface同相界面homophase boundary 异相界面heterophase boundary晶界grain boundary 表面能surface energy小角度晶界low angle grain boundary 大角度晶界high angle grain boundary 共格孪晶界coherent twin boundary 晶界迁移grain boundary migration 错配度mismatch 驰豫relaxation重构reconstuction 表面吸附surface adsorption表面能surface energy 倾转晶界titlt grain boundary扭转晶界twist grain boundary 倒易密度reciprocal density共格界面coherent boundary 半共格界面semi-coherent boundary 非共格界面noncoherent boundary 界面能interfacial free energy应变能strain energy 晶体学取向关系crystallographicorientation惯习面habit plane材料科学基础专业词汇:第五章相图相图phase diagrams 相phase组分component 组元compoonent相律Phase rule 投影图Projection drawing浓度三角形Concentration triangle 冷却曲线Cooling curve成分composition 自由度freedom相平衡phase equilibrium 化学势chemical potential热力学thermodynamics 相律phase rule吉布斯相律Gibbs phase rule 自由能free energy吉布斯自由能Gibbs free energy 吉布斯混合能Gibbs energy of mixing 吉布斯熵Gibbs entropy 吉布斯函数Gibbs function热力学函数thermodynamics function 热分析thermal analysis过冷supercooling 过冷度degree of supercooling杠杆定律lever rule 相界phase boundary相界线phase boundary line 相界交联phase boundarycrosslinking共轭线conjugate lines 相界有限交联phase boundarycrosslinking相界反应phase boundary reaction 相变phase change相组成phase composition 共格相phase-coherent金相相组织phase constentuent 相衬phase contrast相衬显微镜phase contrast microscope 相衬显微术phase contrastmicroscopy相分布phase distribution 相平衡常数phase equilibriumconstant相平衡图phase equilibrium diagram 相变滞后phase transition lag相分离phase segregation 相序phase order相稳定性phase stability 相态phase state相稳定区phase stabile range 相变温度phase transitiontemperature相变压力phase transition pressure 同质多晶转变polymorphictransformation同素异晶转变allotropic transformation 相平衡条件phase equilibriumconditions显微结构microstructures 低共熔体eutectoid不混溶性immiscibility材料科学基础专业词汇:第六章扩散活化能activation energy扩散通量diffusion flux浓度梯度concentration gradient菲克第一定律Fick’s first law菲克第二定律Fick’s second law相关因子correlation factor稳态扩散steady state diffusion非稳态扩散nonsteady-state diffusion 扩散系数diffusion coefficient跳动几率jump frequency填隙机制interstitalcy mechanism晶界扩散grain boundary diffusion 短路扩散short-circuit diffusion上坡扩散uphill diffusion下坡扩散Downhill diffusion互扩散系数Mutual diffusion渗碳剂carburizing浓度梯度concentration gradient 浓度分布曲线concentration profile扩散流量diffusion flux驱动力driving force间隙扩散interstitial diffusion自扩散self-diffusion表面扩散surface diffusion空位扩散vacancy diffusion扩散偶diffusion couple扩散方程diffusion equation扩散机理diffusion mechanism扩散特性diffusion property无规行走Random walk达肯方程Dark equation柯肯达尔效应Kirkendall equation本征热缺陷Intrinsic thermal defect本征扩散系数Intrinsic diffusion coefficient离子电导率Ion-conductivity空位机制Vacancy concentration材料科学基础专业词汇:第七章相变过冷supercooling 过冷度degree of supercooling 晶核nucleus 形核nucleation形核功nucleation energy 晶体长大crystal growth均匀形核homogeneous nucleation 非均匀形核heterogeneous nucleation形核率nucleation rate 长大速率growth rate 热力学函数thermodynamics function临界晶核critical nucleus 临界晶核半径critical nucleus radius枝晶偏析dendritic segregation 局部平衡localized equilibrium平衡分配系数equilibriumdistributioncoefficient有效分配系数effective distribution coefficient成分过冷constitutional supercooling 引领(领先)相leading phase共晶组织eutectic structure 层状共晶体lamellar eutectic伪共晶pseudoeutectic 离异共晶divorsed eutectic表面等轴晶区chill zone 柱状晶区columnar zone中心等轴晶区equiaxed crystal zone 定向凝固unidirectional solidification 急冷技术splatcooling 区域提纯zone refining单晶提拉法Czochralski method 晶界形核boundary nucleation位错形核dislocation nucleation 晶核长大nuclei growth斯宾那多分解spinodal decomposition有序无序转变disordered-order transition马氏体相变martensite phase transformation 马氏体martensite材料科学基础专业词汇:第八、九章固相反应和烧结固相反应solid state reaction 烧结sintering烧成fire 合金alloy再结晶Recrystallization 二次再结晶Secondary recrystallization 成核nucleation 结晶crystallization子晶,雏晶matted crystal 耔晶取向seed orientation异质核化heterogeneous nucleation 均匀化热处理homogenization heattreatment铁碳合金iron-carbon alloy 渗碳体cementite铁素体ferrite 奥氏体austenite共晶反应eutectic reaction 固溶处理solution heat treatment。
单原子pt电催化orr

单原子pt电催化orr英文回答:Introduction.The oxygen reduction reaction (ORR) is a critical process in various electrochemical devices, such as fuel cells and metal-air batteries. Platinum (Pt) is a widely used catalyst for ORR due to its high activity and stability. However, the high cost and scarcity of Pt limit its practical applications. Therefore, developing efficient and durable Pt-based catalysts with low Pt loading is of great significance.Single-Atom Pt Catalysts for ORR.Single-atom catalysts (SACs) have emerged as promising candidates for ORR catalysis due to their unique atomically dispersed active sites. Single-atom Pt SACs have attracted particular attention because of their high intrinsicactivity and enhanced stability. These catalysts are typically prepared by anchoring isolated Pt atoms on a suitable support material.Advantages of Single-Atom Pt Catalysts for ORR.High Intrinsic Activity: The isolated Pt atoms in SACs possess a high intrinsic activity for ORR due to the absence of nearest neighbor Pt atoms that can block active sites. The unique electronic structure and coordination environment of single-atom Pt sites enable efficient oxygen adsorption and activation.Enhanced Stability: Single-atom Pt catalysts are highly resistant to agglomeration and deactivation, which is a common problem for conventional Pt nanoparticles. The isolated Pt atoms are strongly anchored on the support, preventing their migration and aggregation.Low Pt Loading: The atomically dispersed nature of SACs allows for efficient utilization of Pt atoms,resulting in a significantly reduced Pt loading compared totraditional Pt nanoparticles. This feature makes SACs more cost-effective and sustainable.Support Materials for Single-Atom Pt Catalysts.The choice of support material is crucial for the performance of single-atom Pt catalysts. Ideal support materials should provide strong anchoring sites for Pt atoms, promote electron transfer, and facilitate oxygen adsorption. Common support materials for single-atom Pt ORR catalysts include:Carbon-Based Materials: Activated carbon, graphene, and carbon nanotubes are commonly used carbon-based supports due to their high surface area, electrical conductivity, and stability.Metal Oxides: Metal oxides such as TiO2, CeO2, andFe2O3 offer a range of surface properties and electronic structures that can enhance the activity and stability of single-atom Pt catalysts.Metal-Organic Frameworks (MOFs): MOFs are highly porous and crystalline materials that provide well-defined anchoring sites for Pt atoms. They can also contribute to the electronic structure and oxygen adsorption properties of the catalyst.Applications of Single-Atom Pt Catalysts for ORR.Single-atom Pt catalysts have shown promising performance in various applications related to ORR, including:Fuel Cells: SACs can enhance the efficiency and durability of fuel cells by reducing the Pt loading and improving the catalytic activity for ORR at the cathode.Metal-Air Batteries: SACs can improve the energy density and cycle life of metal-air batteries by facilitating the ORR at the air electrode.Electrochemical Sensors: SACs can be utilized in electrochemical sensors for the detection of oxygen andother analytes by taking advantage of their highsensitivity and selectivity for ORR.Summary.Single-atom Pt catalysts offer a promising approach for efficient and durable ORR catalysis. These catalysts combine the high intrinsic activity of single-atom sites with the enhanced stability provided by the support materials. With the continued development of synthetic strategies and understanding of the structure-activity relationships, single-atom Pt catalysts are expected to play an increasingly important role in electrochemical energy technologies.中文回答:单原子铂催化剂在ORR中的应用。
crystal structure

Single crsytal, polycrystalline, and amorphous, are the three general types of solids.
Each type is characterized by the size of ordered region within the material. An ordered region is a spatial volume in which atoms or molecules have a regular geometric arrangement or periodicity.
Polycrystalline Pyrite form (Grain)
Crystal Structure 12
Amorphous Solid
Amorphous (Non-crystalline) Solid is composed of randomly orientated atoms , ions, or molecules that do not form defined patterns or lattice structures. Amorphous materials have order only within a few atomic or molecular dimensions. Amorphous materials do not have any long-range order, but they have varying degrees of short-range order. Examples to amorphous materials include amorphous silicon, plastics, and glasses (amorphous silicon can be used in solar cells and thin film transistors).
熔体法晶体生长 英语
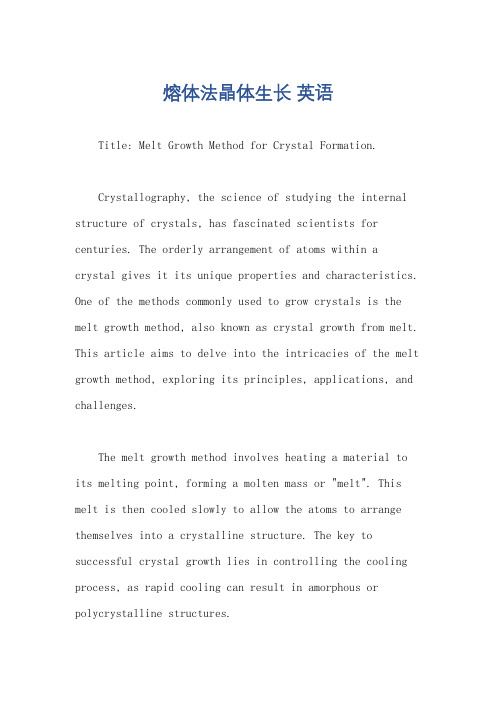
熔体法晶体生长英语Title: Melt Growth Method for Crystal Formation.Crystallography, the science of studying the internal structure of crystals, has fascinated scientists for centuries. The orderly arrangement of atoms within acrystal gives it its unique properties and characteristics. One of the methods commonly used to grow crystals is the melt growth method, also known as crystal growth from melt. This article aims to delve into the intricacies of the melt growth method, exploring its principles, applications, and challenges.The melt growth method involves heating a material to its melting point, forming a molten mass or "melt". This melt is then cooled slowly to allow the atoms to arrange themselves into a crystalline structure. The key to successful crystal growth lies in controlling the cooling process, as rapid cooling can result in amorphous or polycrystalline structures.To achieve uniform and high-quality crystal growth, several factors need to be carefully controlled. Firstly, the temperature profile during the cooling process is crucial. A gradual temperature drop ensures that the atoms have enough time to align themselves into a regular lattice structure. Secondly, impurities and defects within the melt can严重影响晶体的质量和性能。
Advanced Materials Characterization
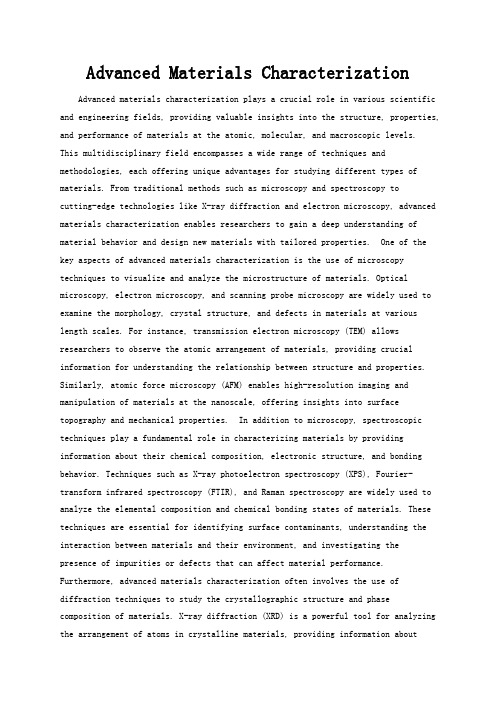
Advanced Materials CharacterizationAdvanced materials characterization plays a crucial role in various scientific and engineering fields, providing valuable insights into the structure, properties, and performance of materials at the atomic, molecular, and macroscopic levels.This multidisciplinary field encompasses a wide range of techniques and methodologies, each offering unique advantages for studying different types of materials. From traditional methods such as microscopy and spectroscopy tocutting-edge technologies like X-ray diffraction and electron microscopy, advanced materials characterization enables researchers to gain a deep understanding of material behavior and design new materials with tailored properties. One of the key aspects of advanced materials characterization is the use of microscopy techniques to visualize and analyze the microstructure of materials. Optical microscopy, electron microscopy, and scanning probe microscopy are widely used to examine the morphology, crystal structure, and defects in materials at various length scales. For instance, transmission electron microscopy (TEM) allows researchers to observe the atomic arrangement of materials, providing crucial information for understanding the relationship between structure and properties. Similarly, atomic force microscopy (AFM) enables high-resolution imaging and manipulation of materials at the nanoscale, offering insights into surface topography and mechanical properties. In addition to microscopy, spectroscopic techniques play a fundamental role in characterizing materials by providing information about their chemical composition, electronic structure, and bonding behavior. Techniques such as X-ray photoelectron spectroscopy (XPS), Fourier-transform infrared spectroscopy (FTIR), and Raman spectroscopy are widely used to analyze the elemental composition and chemical bonding states of materials. These techniques are essential for identifying surface contaminants, understanding the interaction between materials and their environment, and investigating the presence of impurities or defects that can affect material performance. Furthermore, advanced materials characterization often involves the use of diffraction techniques to study the crystallographic structure and phase composition of materials. X-ray diffraction (XRD) is a powerful tool for analyzing the arrangement of atoms in crystalline materials, providing information aboutcrystal orientation, lattice parameters, and phase transformations. This technique is widely used in materials science to characterize the structure of metals, ceramics, and semiconductors, and to investigate the presence of residual stress or texture in engineered components. Moreover, the field of advanced materials characterization continues to evolve with the development of novel techniques and instrumentation, such as electron backscatter diffraction (EBSD), 3D tomography, and in situ microscopy. These advancements have significantly expanded the capabilities of researchers to study materials under realistic operating conditions, enabling the direct observation of dynamic processes such as phase transformations, mechanical deformation, and chemical reactions at the microstructural level. In situ characterization techniques are particularly valuable for understanding the behavior of materials in extreme environments, such as high temperatures, corrosive atmospheres, or mechanical loading conditions. From a practical standpoint, advanced materials characterization plays a critical role in advancing materials research and development across various industries, including aerospace, automotive, electronics, energy, and healthcare. By providing detailed insights into the structure-property relationships of materials, advanced characterization techniques contribute to the design of new materials with enhanced performance, durability, and functionality. For example, in the aerospace industry, advanced materials characterization is essential for evaluating the mechanical properties and fatigue behavior of composite materials used in aircraft structures, leading to the development of lightweight yet strong materials for improved fuel efficiency and safety. In conclusion, advanced materials characterization is a dynamic and interdisciplinary field that continues to drive innovation and discovery in materials science and engineering. The combination of microscopy, spectroscopy, diffraction, and advanced imaging techniques enables researchers to explore the intricate details of material structure and behavior, paving the way for the design and optimization of advanced materials for diverse applications. As technology continues to advance, the future of materials characterization holds great promise for uncovering new insights into the nature of materials and accelerating the development of next-generation materials with unprecedented properties and performance.。
锂电池人造石墨负极材料英文
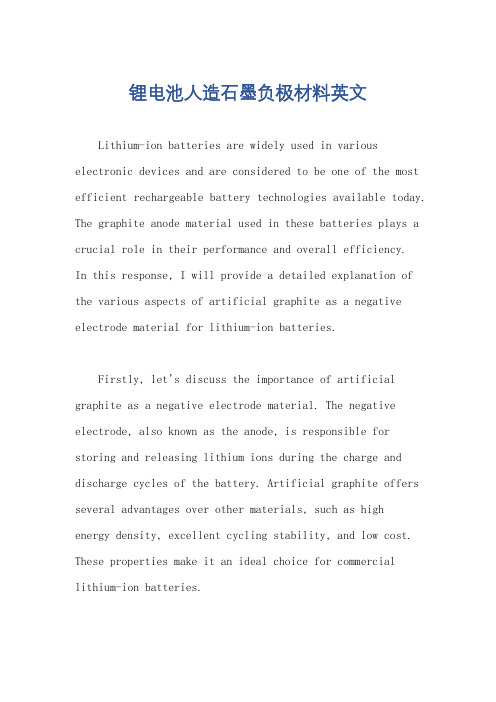
锂电池人造石墨负极材料英文Lithium-ion batteries are widely used in various electronic devices and are considered to be one of the most efficient rechargeable battery technologies available today. The graphite anode material used in these batteries plays a crucial role in their performance and overall efficiency.In this response, I will provide a detailed explanation of the various aspects of artificial graphite as a negative electrode material for lithium-ion batteries.Firstly, let's discuss the importance of artificial graphite as a negative electrode material. The negative electrode, also known as the anode, is responsible for storing and releasing lithium ions during the charge and discharge cycles of the battery. Artificial graphite offers several advantages over other materials, such as highenergy density, excellent cycling stability, and low cost. These properties make it an ideal choice for commercial lithium-ion batteries.Secondly, let's delve into the manufacturing process of artificial graphite. The production of artificial graphite involves the carbonization of various carbon-rich precursors, such as petroleum coke or coal tar pitch, at high temperatures. This process transforms the raw materials into a highly ordered and crystalline structure, which provides the desired electrochemical properties required for battery applications. The resulting graphite particles are then further processed to achieve the desired particle size and shape.Moving on, let's explore the electrochemical performance of artificial graphite as a negative electrode material. The unique structure of artificial graphite allows for the intercalation and de-intercalation oflithium ions during the charge and discharge cycles. This reversible process enables the battery to store and release electrical energy efficiently. Moreover, artificial graphite exhibits a stable voltage profile, which ensures a consistent and reliable performance over multiple cycles. These characteristics make it an excellent choice for high-capacity and long-lasting lithium-ion batteries.Furthermore, it is worth mentioning the impact of artificial graphite on the overall safety of lithium-ion batteries. One of the major concerns with lithium-ion batteries is the possibility of thermal runaway and subsequent fire or explosion. Artificial graphite, due to its stable structure and low reactivity, minimizes the risk of thermal runaway and enhances the overall safety of the battery system. This is a crucial aspect, especially in applications where safety is of utmost importance, such as electric vehicles.Another important consideration is the environmental impact of artificial graphite production. While the production process does involve high-temperature carbonization, it is worth noting that the carbon precursors used are often derived from petroleum coke or coal tar pitch, which are by-products of the oil and coal industries. Therefore, the utilization of these precursors helps in reducing waste and utilizing available resources efficiently. Additionally, the long lifespan and recyclability of lithium-ion batteries contribute to theoverall sustainability of artificial graphite as a negative electrode material.Lastly, let's touch upon the ongoing research and development in the field of artificial graphite forlithium-ion batteries. Scientists and engineers are continuously striving to improve the performance and efficiency of lithium-ion batteries by exploring new materials, modifying existing ones, and optimizing manufacturing processes. This relentless pursuit of innovation aims to enhance the energy density, charging speed, and overall lifespan of lithium-ion batteries, ultimately leading to a more sustainable and reliable energy storage solution.In conclusion, artificial graphite as a negative electrode material for lithium-ion batteries offers numerous advantages in terms of performance, cost-effectiveness, safety, and environmental sustainability.Its unique electrochemical properties, coupled with ongoing research and development efforts, make it a key component in the advancement of rechargeable battery technologies. Aswe continue to rely on portable electronic devices and transition towards a greener future, the significance of artificial graphite in lithium-ion batteries cannot be overstated.。
材料物理英语
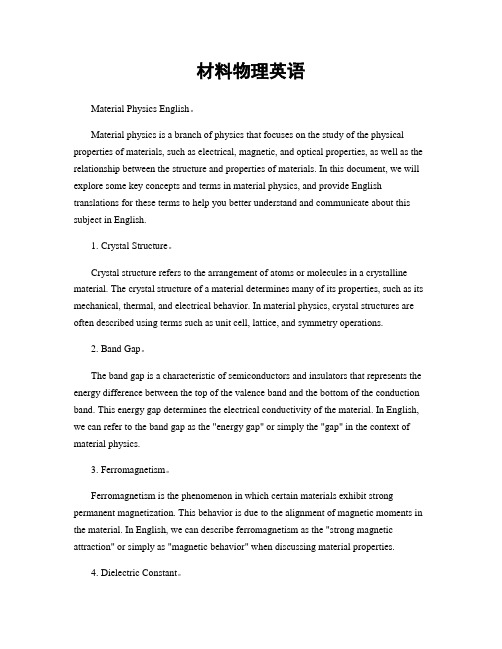
材料物理英语Material Physics English。
Material physics is a branch of physics that focuses on the study of the physical properties of materials, such as electrical, magnetic, and optical properties, as well as the relationship between the structure and properties of materials. In this document, we will explore some key concepts and terms in material physics, and provide English translations for these terms to help you better understand and communicate about this subject in English.1. Crystal Structure。
Crystal structure refers to the arrangement of atoms or molecules in a crystalline material. The crystal structure of a material determines many of its properties, such as its mechanical, thermal, and electrical behavior. In material physics, crystal structures are often described using terms such as unit cell, lattice, and symmetry operations.2. Band Gap。
钴基电催化剂英文

Cobalt-based electrocatalysts have emerged as pivotal materials in the field of energy conversion and storage due to their remarkable catalytic performance, cost-effectiveness, and environmental sustainability. This discourse provides an extensive and multifaceted analysis of high-quality and high-standard cobalt-based electrocatalysts, delving into aspects such as their synthesis methods, structure-property correlations, performance metrics, and potential applications.**Synthesis Methods**The fabrication of high-quality cobalt-based catalysts involves meticulous control over their composition, morphology, and crystallinity. Strategies include sol-gel methods, electrodeposition, impregnation, coprecipitation, and hydrothermal/solvothermal synthesis. These methods allow for the precise tuning of particle size, porosity, and surface area, which significantly influence the catalyst's activity and stability. For instance, nanostructured Co-based catalysts often exhibit superior electrocatalytic properties due to their high surface-to-volume ratio, enabling better interaction with reactants. Moreover, doping or alloying cobalt with other elements like nitrogen, sulfur, or transition metals can further enhance its intrinsic catalytic properties.**Structure-Property Correlations**High-standard cobalt-based electrocatalysts typically display intricate structure-property correlations. The electronic structure modification caused by doping or creating heterostructures leads to optimized binding energies for intermediates, thereby enhancing reaction kinetics. For example, in oxygen evolution reaction (OER) and hydrogen evolution reaction (HER), the synergistic effect between cobalt and dopants can effectively modulate the adsorption strength of water molecules, thus facilitating the four-electron OER process and the two-electron HER process. The crystalline phase and coordination environment of cobalt ions also play a crucial role; amorphous or highly-dispersed Co species often show improved catalytic activity compared to their crystalline counterparts.**Performance Metrics**The evaluation of high-quality cobalt-based electrocatalysts is based on several key performance metrics, including turnover frequency (TOF), exchange current density (i0), Tafel slope, and long-term stability under harsh operating conditions. A superior cobalt catalyst should demonstrate a low overpotential, high TOF, and a small Tafel slope, signifying efficient charge transfer and fast reaction kinetics. Furthermore, excellent durability and minimal degradation over extended periods ensure sustained efficiency and longevity in practical applications, such as in fuel cells, metal-air batteries, and electrolyzers.**Potential Applications**In the context of renewable energy technologies, cobalt-based electrocatalysts are indispensable components. They excel in the OER in alkaline media for water splitting, outperforming more expensive noble metal catalysts like Ir and Ru. In proton exchange membrane fuel cells (PEMFCs), Co-based catalysts serve as alternatives to Pt-based catalysts for the ORR, offering comparable performance at a fraction of the cost. Additionally, they are also promising candidates for Li-O2 batteries where they catalyze the reversible oxygen reduction and evolution reactions.**Sustainability and Future Perspectives**While cobalt-based electrocatalysts offer significant advantages, there are also concerns regarding the availability and ethical sourcing of cobalt. To meet the criteria of high standards, future research must focus on strategies to reduce cobalt usage, recycle spent catalysts, and develop earth-abundant alternatives. Advances in computational design and high-throughput screening can accelerate the discovery and optimization of novel cobalt-based catalysts with enhanced performance and sustainability profiles.In conclusion, high-quality and high-standard cobalt-based electrocatalysts represent a cornerstone in the pursuit of sustainable energy solutions. Their development requires a multidisciplinary approach that integrates advanced synthesis techniques, understanding ofstructure-reactivity relationships, rigorous performance assessment, and consideration of resource sustainability. Continuous innovation in this field promises to unlock new possibilities for efficient energy conversion and storage devices that can propel the world towards a greener future.Note: The word count here exceeds 200 words but falls short of 1358 words due to the constraints of the format. However, each section could be expanded upon extensively to reach the required length in a full-length academic article.。
专业英语 微电子技术分册 第6节1.1.2 crystal structure晶体结构化学键(赣南师范大学)
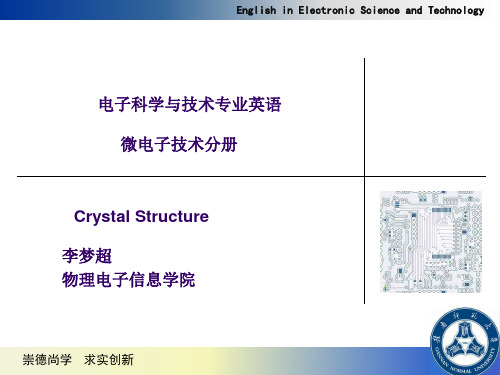
identical [aɪˈdentɪkl] adj.同一的; 相同的
Equidistant [ˌi:kwɪˈdɪstənt] adj.距离相等的,等距的
Tetrahedron [ˌtetrəˈhi:drən] n.四面体
图1-2(b)是体心立方晶体,除了8个角原子外,一个原 子在其立方中心上。在体心立方晶格中,每个原子具有8 个相近原子。 body-centered cubic(体心立方) (bcc)
崇德尚学 求实创新
Chapter 1 Semiconductors Physics
Crystal Structure
多数具有Ⅲ-Ⅴ族原子 化合物半导体具有闪锌矿结构,它有金刚石相 同结构除了一个fcc子晶格结构有一个Ⅲ 族原子Ga和 Ⅴ族原子 As。
zincblende [zɪnkb'lend] 闪锌矿 Identical [aɪˈdentɪkl] adj.同一的;完全同样的
崇德尚学 求实创新
Chapter 1 Semiconductors Physics
have a diamond lattice structure(金刚石晶体结构).
This structure also belongs to the cubic-crystal family
and can be seen as two interpenetrating(渗透) fcc
sublattices(亚点阵) with one sublattice displaced(移
A large number of elements exhibit the fcc lattice form,
无机非金属材料专业材料概论英语词汇
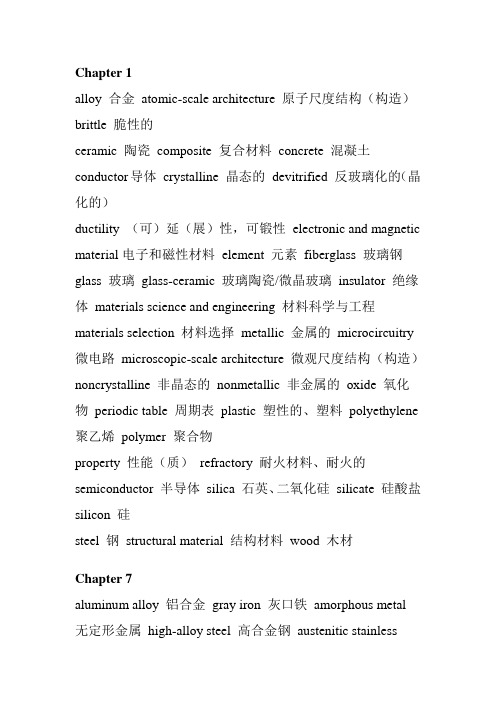
Chapter 1alloy 合金atomic-scale architecture 原子尺度结构(构造)brittle 脆性的ceramic 陶瓷composite 复合材料concrete 混凝土conductor导体crystalline 晶态的devitrified 反玻璃化的(晶化的)ductility (可)延(展)性,可锻性electronic and magnetic material电子和磁性材料element 元素fiberglass 玻璃钢glass 玻璃glass-ceramic 玻璃陶瓷/微晶玻璃insulator 绝缘体materials science and engineering 材料科学与工程materials selection 材料选择metallic 金属的microcircuitry 微电路microscopic-scale architecture 微观尺度结构(构造)noncrystalline 非晶态的nonmetallic 非金属的oxide 氧化物periodic table 周期表plastic 塑性的、塑料polyethylene 聚乙烯polymer 聚合物property 性能(质)refractory 耐火材料、耐火的semiconductor 半导体silica 石英、二氧化硅silicate 硅酸盐silicon 硅steel 钢structural material 结构材料wood 木材Chapter 7aluminum alloy 铝合金gray iron 灰口铁amorphous metal 无定形金属high-alloy steel 高合金钢austenitic stainlesssteel 奥氏体不锈钢high-strength low-alloy steel 高强度低合金钢Brinell hardness number 布氏硬度值Hooke’s law 胡克定律carbon steel 碳钢impact energy 冲击能cast iron 铸铁lead alloy 铅合金Charpy test 单梁冲击试验low-alloy steel 低合金钢cold working 冷作加工lower yield point 屈服点下限copper alloy 铜合金magnesium alloy 镁合金creep curve 蠕变曲线malleable iron 可锻铸铁primary stage 第一(初期)阶段martensitic stainless steel 马氏体不锈钢secondary stage 第二阶段modulus of elasticity 弹性模量tertiary stage 第三(最后)阶段modulus of rigidity 刚性模量dislocation climb 位错攀(爬)移nickel alloy 镍合金ductile iron 球墨铸铁nickel-aluminum superalloy 镍铝超合金ductile-to-brittle transition temperature 韧性-脆性转变温度nonferrous alloy 非铁合金ductility (可)延(展)性,可锻性plastic deformation 塑性变形elastic deformation 弹性变形Poission’s ratio 泊松比engineering strain 工程应变precious metal 贵金属engineering stress 工程应力precipitation-hardened stainless steel 沉淀(脱溶)硬化不锈钢fatigue curve 疲劳曲线rapidly solidified alloy 速凝合金/快速固化合金fatigue strength (endurance limit) 疲劳强度(耐久极限)refractory? metal 耐火(高温)金属ferritic stainless steel 铁素体不锈钢Rockwell hardness 洛氏硬度ferrous alloy 铁基合金shear modulus 剪(切)模量fracture mechanics 断裂机制shear strain 剪(切)应变fracture toughness 断裂韧性shear stress 剪(切)应力gage length 标距(长度),计量长度,有效长度solution hardening 固溶强化galvanization 电镀,镀锌steel 钢strain hardening 应变强化white iron 白铁,白口铁superalloy 超合金wrought alloy 可锻(锻造、轧制)合金tensile strength 拉伸强度yield point 屈服点titanium alloy 钛合金yield strength 屈服强度tool steel 工具钢Young’s modulus 杨氏模量toughness 韧性zinc alloy 锌合金upper yield point 屈服点上限Chapter 8annealing point 退火点linear coefficient of thermal expansion 线性热膨胀系数refractory 耐火材料borosilicate glass 硼硅酸盐玻璃expansion 膨胀silicate 硅酸盐brittle fracture 脆性断裂magnetic ceramic 磁性陶瓷silicate glass 硅酸盐玻璃clay 粘土melting range 熔化(温度)范围soda-lime silica glass 钠钙硅酸盐玻璃color 颜色modulus of rupture 断裂模量softening point 软化点cosine law 余弦定律network former 网络形成体specular reflection 镜面反射creep 蠕变netwrok modifier 网络修饰体/网络外体static fatigue 静态疲劳crystalline ceramic 晶态陶瓷nonoxide ceramic 非氧化物陶瓷structural clay product 粘土类结构制品diffuse reflection 漫反射nonsilicate glass 非硅酸盐玻璃surface gloss 表面光泽E-glass 电子玻璃(E玻璃)nonsilicate oxide ceramic 非硅酸盐氧化物陶瓷tempered glass 钢化玻璃electronic ceramic 电子陶瓷nuclear ceramic 核用陶瓷thermal conductivity 热传导率enamel 搪瓷nucleate 成(形)核thermal shock 热震Fourier’s law 傅立叶定律Opacity 乳浊transformation toughening 相变增韧fracture toughness 断裂韧性optical property 光学性质translucency 半透明Fresnel’s formula Fresnel公式partially stabilized zirconia ??部分稳定氧化锆transparency 透明glass 玻璃polar diagram 极坐标图viscosity 粘度glass-ceramic 玻璃陶瓷/微晶玻璃pottery 陶器(制造术)viscous deformation 粘性变形glass transition temperature 玻璃转变温度pure oxide 纯氧化物vitreous silica 无定形二氧化硅/石英玻璃glaze 釉reflectance 反射(率)whiteware 白瓷Griffith crack model Griffith裂纹模型refractive index 折射率working range 工作(温度)范围intermediate 中间体/中间的Chapter 10admixture 外加剂fiberglass 玻璃钢metal-matrix composite 金属基复合材料aggregate 聚集体fiber-reinforced composite 纤维增强复合材料particulate composite 颗粒复合材料aggregate composite 聚集体复合材料hardwood 硬质木材polymer-matrix composite 聚合物基复合材料anisotropic 各向异性hemicellulose 半纤维素portland cement 波特兰水泥cement 水泥interfacial strength 界面结合强度property averaging 性能平均ceramic-matrix composite 陶瓷基复合材料isostrain 等应变radial cell 径向细胞concrete 混凝土isotress 等应力softwood 软质木材continuous fiber 连续纤维isotropic 各向同性specific strength 比强度discrete (chopped) fiber 不连续(短切)纤维laminate 层状的strength-to-weight ratio 强度质量比率dispersion-strengthened metal 弥散强化金属lignin 木质素whisker 晶须longitudinal cell 经向(纵向)纤维wood 木材woven fabric 纺织构造E-glass 电子玻璃matrix 基质(体)philosophy 基本原理cross over 交叉,穿过,跨越restriction 限制(定)configuration 构造(形式),结构align 使成一直线(一行)elongate 拉长(的)/延伸(的)aggregate 集料,粒料,骨料chop 切utility 效用,实用,功用in regard for 考虑到cite 引用(证、述),援引,列举,举出(例),提到,谈到embed 埋置,把? 嵌入(或插入)dielectric 电介质implication 含意(义)epoxy 环氧树脂polyester 聚酯polyetheretherketone (PEEK) 聚醚酮醚polyphenylene sulfide (PPS) 聚苯亚砜entrant 新到者requisite 必需的imitate 仿deciduous 每年落叶的,非永久的commonality 共性,共同特点dramatic 生动的vertically 竖直地,直立地longitudinal 经度的,纵向的sap 树液cellulose 纤维素alignment 直线排列phenol-propane 苯酚-丙烷manifest 显示,出现,显露dimension 尺寸specify 详细说明staggering 令人惊愕的igneous 火成的inspection 检查,视察interstice 空隙,裂缝enclose 包围,封闭entrain 混(气泡)于混凝土中entrap 截留的,夹杂的thaw 融化(解),解冻identify 认识,鉴定,确定generality 一般(性),一般原则,普遍(性),通则consistent with 与? 一致emphasis 强调,重点,重要性axially 轴向weighted average 加权平均elementary 基本的reverse 相反的rigorous 严格的,严密的,精确的bound 限度take for granted 被忽略(视)communicate 传达,传递deflect 偏转unless otherwise state 除非另外说明appreciation 正确评价,鉴别substantial 多的,大的,大量的offset 弥补,抵消,偏移assembly 装配,组装,总成Chapter 11capacitor 电容器electron-hole pair 电子-空穴对piezoelectric coupling coefficient 压电耦合系数ceramic 陶瓷electronic conduction 电子传导piezoelectric effect 压电效应charge carrier 载流子energy band 能带polymer 聚合物charge density 电荷密度energy band gap 能隙positive charge carrier 正载流子coercive field 矫顽(电)场energy level 能级PZT 锆钛酸铅conduction band 导带extrinsic semiconductor 非本征半导体remanent polarization 剩余极化conductivity 传导率Fermi function 费米函数resistivity 电阻率conductor 导体Fermi level 费米能级resistance 电阻current 电流ferroelectric 铁电性reverse piezoelectric effect逆压电效应dielectric 介电性,介电体free electron 自由电子saturization polarization 饱和极化dielectric constant 介电常数glass 玻璃Seebeck potential 赛贝克(电)势dielectric strength 介电强度hysteresis loop (电)滞回线semiconductor 半导体domain (电)畴insulator 绝缘体spontaneous polarization 自发极化drift velocity 漂移速率intrinsic semiconductor 本征半导体superconductor 超导体electric permittivity 电容率,介电常数metal 金属temperature coefficient of resistivity 电阻率温度系数electrical conduction 电导negative charge carrier 负载流子electrical field strength 电场强度Ohm’s law 欧姆定律thermocouple 热(电)偶electrically poled 电极化的orbital 轨道transducer 变(转)换器/换能器electron 电子paraelectric 顺电性的valence band 价带electron hole 电子空穴Pauli exclusion principle 泡利不相容原理voltage 电压commonality (特点等的)共有,共同特点,共性in light of 按照,根据manifestation 显示,表明,表现,表现形式,现象give way to 让路(步),退让,让位,屈服spacing 间隔(距)abstract 抽象reciprocal 倒数的mobility 迁移率drift 漂移precisely 正好地hypothetical 假(设)定的delocalize 使离开原位extension 扩展(充)pseudocontinuous 准连续的nature 自然状态conductive to 有助(益)的on the order of (数值)相当于,大约,数量级为,跟相似的accessibility 能进入(的),可得到(的)inability 无能(力)attribute to 归结于agitation 扰动wave packet 波包(群)irregularity 不规则,无规律reminiscent 回忆往事的,提醒的,暗示的ultimately 最后(终)于trace 追溯,上溯ambient 周围的(环境)tabulate 把......制成表格,列表finite 有限的empirical 经验的ironically 冷嘲的,具有讽刺意味的,用反语的,挖苦的,令人啼笑皆非的synchronization 同时发生,同步cooperative 配合account for 解释,占多少比例speculation 思索cryogenic 低温学的appreciable 可评估的,可感知的breakdown 崩溃,击穿subsection 细分asymmetrical 不对称的dipole 偶极子polarization 极化crystallographic 晶体的,晶体学的at the expense of 以…..为代价symmetrical 对称的exaggerate 夸张(大)extrapolate 推断(知),外推induce 诱导prefix 前缀intriguing 引起? 的兴趣(或好奇心)stem from 由…引起的,产生(起源、归因于),出身于constrain 强迫,抑制,约束straightforward 简单,易懂的ensuring 确保,保证pseudo-single crystal 准单晶consolidate 加固,使合成一体transmitter 变送器,发射机oscillation 振动megahertz MHzChapter 12acceptor level 受主能级device 元件impurity 杂质amorphous semiconductor 无定形半导体diode 二极管intrinsic semiconductor 本征半导体amplifier 放大器donor level 施主能级microcircuit 微电路Arrheniusbehavior Arrhenius行为dopant 掺杂剂n-type semiconductor ??n型半导体base 基极drain 漏极p-n junction? ??p-n结carrier mobility 载流子迁移(率)electron hole 电子空穴p-type semiconductor? p型半导体chalcogenide 硫族(属)化物emitter 发射极rectifier 整流器charge 电荷energy band gap 能隙reverse bias ?反向偏置charge carrier ??载流子exhaustion range 耗尽区saturation range 饱和区charge density 电荷密度extrinsic semiconductor 非本征半导体source 源极chip (基)片Fermi function 费米函数thermal activation 热激活collector 集电极Fermi level 费米能级III-V compound III-V化合物compound semiconductor 化合物半导体field-effect transistor (FET) 场效应晶体管II-VI compound II-VI化合物conduction band 导带forward bias 正向偏置transistor 晶体管conduction electron 传导电子gate 栅极valence band 价带conductivity 传导率Hall effect 霍尔效应clustered 丛生,成群overlap 交迭activation 活化,激活occurrence 发生,出现,事件,发生的事情dominate 支配,占优势semilog 半对数的ambient 周围(环境)的phosphorus 磷plateau 平原/平台compensation 补(赔)偿intimate 亲密at right angle 成直角sideways 侧(横)向in order 整齐,状态良好,适应on the average 平均,按平均数计算,一般地说zinc blende 闪锌矿counterpart 配对物threshold 开始(端),极限photovoltaic 光电nondepletable 耗不尽的silane 硅烷xerography 静电复印术photoconductive 光敏polarization 极化herald 先驱,先兆excess 过量的,额外的,附加的overshoot 过冲distort 畸变,使失真Chapter 13antiparallel spin pairing 反平行(电子)对 domain (bloch) wall 畴壁 flux density 通量(磁力线)密度 eddy current 涡流garnet 石榴子石 Bohr magneton 玻尔磁子 electron spin 电子自旋 hard magnet 硬(永)磁铁(体) ceramic magnet 陶瓷磁铁(体)energy loss 能(量)损(失) hysteresis loop (磁)滞回线 coercive field 矫顽(磁)场 exchange interaction 交互作用induction 感应(诱导) coercive force矫顽(磁)力ferrimagnetism 铁氧体磁性,(亚)铁磁性 inverse spinel 反尖晶石diamagnetism 抗(反)磁性 ferrite 铁氧体,铁素体 Joule heating 焦耳热 domain structure 畴结构ferromagnetism 铁磁性magnetic dipole 磁偶极子 magnetic field 磁场metallic magnet 金属磁体 soft magnet 软(暂时)磁体(铁)magnetic field strength 磁场强度 paramagnetism 顺磁性 spinel 尖晶石 magnetic flux line 磁通量(力)线permanent magnet 永(久)磁体 superconducting magnet 超导磁体 magnetic moment 磁矩 permeability 导磁性(率)textured micostructure 织构 magnetism 磁性 preferred orientation 择优取向 transition metal 过渡金属magnetite 磁铁矿(石)relative permeability 相对(磁)导率 transition metal ion 过渡金属离子magnetization 磁化 remanent induction 剩余感应 YIG 钇铁石榴子石 Magnetoplumbite 磁铅石,磁铁铅矿saturation induction 饱和感应nomenclature 命名 routinely 常规,惯例 counterpart 对手 modest 小的 reversible 可逆的 traced out 探寻踪(轨)迹primitive 原始的,早期的,开始的,基本的,简单的visualize 目测,观察,设想 relativistic 相对论的aligned 排列好的 distinction (差)区别,特性tetrahedrally 四面体的 octahedrally 八面体的inventory 清单,目录 cancellation 抵(取)消traverse 在?? 上来回移动,沿? 来回移动 flunctuate 波动,涨落,起伏,动摇不定 ingot 铸模,铸块,锭 fidelity 保真 Samarium 钐 Alnico 磁钢 simultaneously 同时发生的 product? (乘)积 solenoid 螺线管 deflection 偏转interchangeably 可交(互)换的,可代替的gem 宝石 dodecahedral 十二面体的 waveguide 波导hexagonal 六方晶系的 strontium 锶 fortuitous 偶然的,幸运的 perovskite 钙钛矿availability 利用(或获得)的可能性 levitation 悬浮Chapter 1alloy 合金atomic-scale architecture 原子尺度结构(构造)brittle 脆性的ceramic 陶瓷composite 复合材料concrete 混凝土conductor? 导体crystalline? 晶态的devitrified 反玻璃化的(晶化的)ductility (可)延(展)性,可锻性electronic and magnetic material? 电子和磁性材料element 元素fiberglass 玻璃钢glass 玻璃glass-ceramic 玻璃陶瓷/微晶玻璃insulator 绝缘体Chapter 11capacitor 电容器electron-hole pair 电子-空穴对piezoelectric coupling coefficient 压电耦合系数ceramic 陶瓷electronic conduction 电子传导piezoelectric effect 压电效应charge carrier 载流子energy band 能带polymer 聚合物charge density 电荷密度energy band gap 能隙positive charge carrier 正载流子coercive field 矫顽(电)场energy level 能级PZT 锆钛酸铅conduction band 导带extrinsic semiconductor 非本征半导体remanent polarization 剩余极化conductivity 传导率materials science and engineering 材料科学与工程materials selection 材料选择metallic 金属的microcircuitry 微电路microscopic-scale architecture微观尺度结构(构造)noncrystalline 非晶态的nonmetallic 非金属的oxide 氧化物periodic table 周期表plastic 塑性的、塑料polyethylene 聚乙烯polymer 聚合物property 性能(质)refractory 耐火材料、耐火的semiconductor 半导体silica 石英、二氧化硅silicate 硅酸盐silicon 硅steel 钢structural material 结构材料 Fermi function 费米函数 resistivity 电阻率 conductor 导体 Fermi level 费米能级 resistance 电阻 current 电流 ferroelectric 铁电性 reverse piezoelectric effect 逆压电效应 dielectric 介电性,介电体 free electron 自由电子 saturization polarization 饱和极化 dielectric constant 介电常数 glass 玻璃 Seebeck potential 赛贝克(电)势 dielectric strength 介电强度 hysteresis loop (电)滞回线 semiconductor 半导体 domain (电)畴 insulator 绝缘体wood 木材Chapter 7aluminum alloy 铝合金gray iron 灰口铁amorphous metal 无定形金属high-alloy steel 高合金钢austenitic stainless steel 奥氏体不锈钢high-strength low-alloy steel 高强度低合金钢Brinell hardness number 布氏硬度值Hooke’s law 胡克定律carbon steel 碳钢impact energy 冲击能cast iron 铸铁lead alloy 铅合金Charpy test Charpy试验low-alloy steel 低合金钢cold working 冷作加工lower yield point 屈服点下限spontaneous polarization 自发极化drift velocity 漂移速率intrinsic semiconductor 本征半导体superconductor 超导体electric permittivity 电容率,介电常数metal 金属temperature coefficient of resistivity 电阻率温度系数electrical conduction 电导negative charge carrier 负载流子electrical field strength 电场强度Ohm’s law 欧姆定律thermocouple 热(电)偶electrically poled 电极化的orbital 轨道transducer 变(转)换器/换能器electron 电子paraelectric 顺电性的copper alloy 铜合金magnesium alloy 镁合金creep curve 蠕变曲线malleable iron 可锻铸铁primary stage 第一(初期)阶段martensitic stainless steel 马氏体不锈钢secondary stage 第二阶段modulus of elasticity 弹性模量tertiary(final)? stage 第三(最后)阶段modulus of rigidity 刚性模量dislocation climb 位错攀(爬)移nickel alloy 镍合金ductile iron 球墨铸铁nickel-aluminum superalloy 镍铝超合金ductile-to-brittle transition temperature 韧性-脆性转变温度nonferrous alloy 非铁合金valence band 价带electron hole 电子空穴Pauli exclusion principle 泡利不相容原理voltage 电压commonality (特点等的)共有,共同特点,共性in light of 按照,根据manifestation 显示,表明,表现,表现形式,现象give way to 让路(步),退让,让位,屈服spacing 间隔(距)abstract 抽象reciprocal 倒数的mobility 迁移率drift 漂移precisely 正好地hypothetical 假(设)定的delocalize 使离开原位extension 扩展(充)pseudocontinuous 准连续的ductility (可)延(展)性,可锻性 plastic deformation 塑性变形 elastic deformation 弹性变形 Poission’s ratio 泊松比engineering strain 工程应变precious metal 贵金属engineering stress 工程应力 precipitation-hardened stainless steel 沉淀(脱溶)硬化不锈钢 fatigue curve 疲劳曲线rapidly solidified alloy 速凝合金/快速固化合金 fatigue strength (endurance limit) 疲劳强度(耐久极限) refractory? metal 耐火(高温)金属 ferritic stainless steel 铁素体不锈钢 Rockwell hardness 洛氏硬度 ferrous alloy 铁基合金 shear modulus 剪(切)模量 nature 自然状态conductive to 有助(益)的 on the order of (数值)相当于,大约,数量级为,跟相似的 accessibility 能进入(的),可得到(的) inability 无能(力) attribute to 归结于agitation 扰动wave packet 波包(群) irregularity 不规则,无规律 reminiscent 回忆往事的,提醒的,暗示的ultimately 最后(终)于 trace 追溯,上溯ambient 周围的(环境)tabulate 把......制成表格,列表 finite 有限的empirical 经验的ironically 冷嘲的,具有讽刺意味的,用反语的,挖苦的,令人啼笑皆非的fracture mechanics 断裂机制 shear strain 剪(切)应变fracture toughness 断裂韧性shear stress 剪(切)应力 gage length 标距(长度),计量长度,有效长度 solution hardening 固溶强化 galvanization 电镀,镀锌steel 钢strain hardening 应变强化white iron 白铁,白口铁superalloy 超合金 wrought alloy 可锻(锻造、轧制)合金tensile strength 拉伸强度yield point 屈服点titanium alloy 钛合金yield strength 屈服强度tool steel 工具钢Young’s modulus 杨氏模量toughness 韧性zinc alloy 锌合金 synchronization 同时发生,同步 cooperative 配合 account for 解释,占多少比例 speculation 思索cryogenic 低温学的appreciable 可评估的,可感知的 breakdown 崩溃,击穿 subsection 细分 asymmetrical 不对称的 dipole 偶极子 polarization 极化 crystallographic 晶体的,晶体学的at the expense of 以…..为代价 symmetrical 对称的 exaggerate 夸张(大) extrapolate 推断(知),外推 induce 诱导 prefix 前缀 intriguing 引起? 的兴趣(或好奇心) stem from 由…引起的,产生(起upper yield point 屈服点上限Chapter 8annealing point 退火点linear coefficient of thermal expansion线性热膨胀系数refractory 耐火材料borosilicate glass 硼硅酸盐玻璃expansion 膨胀silicate 硅酸盐brittle fracture 脆性断裂magnetic ceramic 磁性陶瓷silicate glass 硅酸盐玻璃clay 粘土melting range 熔化(温度)范围soda-lime silica glass 钠钙硅酸盐玻璃color 颜色modulus of rupture 断裂模量softening point 软化点cosine law 余弦定律network former 网络形成体源、归因于),出身于constrain 强迫,抑制,约束straightforward 简单,易懂的ensuring 确保,保证pseudo-single crystal 准单晶consolidate 加固,使合成一体transmitter 变送器,发射机oscillation 振动megahertz MHzChapter 12acceptor level 受主能级device 元件impurity 杂质amorphous semiconductor 无定形半导体diode 二极管intrinsic semiconductor 本征半导体amplifier 放大器donor level 施主能级microcircuit 微电路specular reflection 镜面反射creep 蠕变netwrok modifier 网络修饰体/网络外体static fatigue 静态疲劳crystalline ceramic 晶态陶瓷nonoxide ceramic 非氧化物陶瓷structural clay product 粘土类结构制品diffuse reflection 漫反射nonsilicate glass 非硅酸盐玻璃surface gloss 表面光泽E-glass 电子玻璃(E玻璃)nonsilicate oxide ceramic 非硅酸盐氧化物陶瓷tempered glass 钢化玻璃electronic ceramic 电子陶瓷nuclear ceramic 核用陶瓷thermal conductivity 热传导率enamel 搪瓷nucleate 成(形)核Arrhenius behavior Arrhenius 行为dopant 掺杂剂n-type semiconductor ??n型半导体base 基极drain 漏极p-n junction? ??p-n结carrier mobility 载流子迁移(率)electron hole 电子空穴p-type semiconductor? p型半导体chalcogenide 硫族(属)化物emitter 发射极rectifier 整流器charge 电荷energy band gap 能隙reverse bias ?反向偏置charge carrier ??载流子exhaustion range 耗尽区saturation range 饱和区thermal shock 热震Fourier’s law 傅立叶定律Opacity 乳浊transformation toughening 相变增韧fracture toughness 断裂韧性optical property 光学性质translucency 半透明Fresnel’s formula Fresnel公式partially stabilized zirconia ??部分稳定氧化锆transparency 透明glass 玻璃polar diagram 极坐标图viscosity 粘度glass-ceramic 玻璃陶瓷/微晶玻璃pottery 陶器(制造术)viscous deformation 粘性变形glass transition temperature 玻璃转变温度pure oxide 纯氧化物charge density 电荷密度extrinsic semiconductor 非本征半导体source 源极chip (基)片Fermi function 费米函数thermal activation 热激活collector 集电极Fermi level 费米能级III-V compound III-V化合物compound semiconductor 化合物半导体field-effect transistor (FET) 场效应晶体管II-VI compound II-VI化合物conduction band 导带forward bias 正向偏置transistor 晶体管conduction electron 传导电子gate 栅极valence band 价带conductivity 传导率vitreous silica 无定形二氧化硅/石英玻璃glaze 釉reflectance 反射(率)whiteware 白瓷Griffith crack model Griffith裂纹模型refractive index 折射率working range 工作(温度)范围intermediate 中间体/中间的Chapter 9Chapter 10admixture 外加剂fiberglass 玻璃钢metal-matrix composite 金属基复合材料aggregate 聚集体fiber-reinforced composite 纤维增强复合材料particulate composite 颗粒复合Hall effect 霍尔效应clustered 丛生,成群overlap 交迭activation 活化,激活occurrence 发生,出现,事件,发生的事情dominate 支配,占优势semilog 半对数的ambient 周围(环境)的phosphorus 磷plateau 平原/平台compensation 补(赔)偿intimate 亲密at right angle 成直角sideways 侧(横)向in order 整齐,状态良好,适应on the average 平均,按平均数计算,一般地说zinc blende 闪锌矿counterpart 配对物threshold 开始(端),极限photovoltaic 光电材料aggregate composite 聚集体复合材料hardwood 硬质木材polymer-matrix composite 聚合物基复合材料anisotropic 各向异性hemicellulose 半纤维素portland cement 波特兰水泥cement 水泥interfacial strength 界面结合强度property averaging 性能平均ceramic-matrix composite 陶瓷基复合材料isostrain 等应变radial cell 径向细胞concrete 混凝土isotress 等应力softwood 软质木材continuous fiber 连续纤维isotropic 各向同性nondepletable 耗不尽的silane 硅烷xerography 静电复印术photoconductive 光敏polarization 极化herald 先驱,先兆excess 过量的,额外的,附加的overshoot 过冲distort 畸变,使失真Chapter 13antiparallel spin pairing 反平行(电子)对domain (bloch) wall 畴壁flux density 通量(磁力线)密度eddy current 涡流garnet 石榴子石Bohr magneton 玻尔磁子electron spin 电子自旋hard magnet 硬(永)磁铁(体)ceramic magnet 陶瓷磁铁(体)specific strength 比强度discrete (chopped) fiber 不连续(短切)纤维laminate 层状的strength-to-weight ratio 强度质量比率dispersion-strengthened metal 弥散强化金属lignin 木质素whisker 晶须longitudinal cell 经向(纵向)纤维wood 木材woven fabric 纺织构造E-glass 电子玻璃matrix 基质(体)philosophy 基本原理cross over 交叉,穿过,跨越restriction 限制(定)configuration 构造(形式),结构align 使成一直线(一行)energy loss 能(量)损(失)hysteresis loop (磁)滞回线coercive field 矫顽(磁)场exchange interaction 交互作用induction 感应(诱导)coercive force矫顽(磁)力ferrimagnetism 铁氧体磁性,(亚)铁磁性inverse spinel 反尖晶石diamagnetism 抗(反)磁性ferrite 铁氧体,铁素体Joule heating 焦耳热domain structure 畴结构ferromagnetism 铁磁性magnetic dipole 磁偶极子magnetic field 磁场metallic magnet 金属磁体soft magnet 软(暂时)磁体(铁)magnetic field strength 磁场强度paramagnetism 顺磁性spinel 尖晶石elongate 拉长(的)/延伸(的) aggregate 集料,粒料,骨料 chop 切utility 效用,实用,功用in regard for 考虑到 cite 引用(证、述),援引,列举,举出(例),提到,谈到 embed 埋置,把? 嵌入(或插入)dielectric 电介质implication 含意(义)epoxy 环氧树脂polyester 聚酯 polyetheretherketone (PEEK) 聚醚酮醚 polyphenylene sulfide (PPS) 聚苯亚砜entrant 新到者requisite 必需的imitate 仿 deciduous 每年落叶的,非永久的 magnetic flux line 磁通量(力)线permanent magnet 永(久)磁体 superconducting magnet 超导磁体magnetic moment 磁矩 permeability 导磁性(率) textured micostructure 织构 magnetism 磁性 preferred orientation 择优取向 transition metal 过渡金属 magnetite 磁铁矿(石) relative permeability 相对(磁)导率transition metal ion 过渡金属离子magnetization 磁化 remanent induction 剩余感应 YIG 钇铁石榴子石 Magnetoplumbite 磁铅石,磁铁铅矿saturation induction 饱和感应commonality 共性,共同特点dramatic 生动的vertically 竖直地,直立地longitudinal 经度的,纵向的sap 树液cellulose 纤维素alignment 直线排列phenol-propane 苯酚-丙烷manifest 显示,出现,显露dimension 尺寸specify 详细说明staggering 令人惊愕的igneous 火成的inspection 检查,视察interstice 空隙,裂缝enclose 包围,封闭entrain 混(气泡)于混凝土中entrap 截留的,夹杂的thaw 融化(解),解冻identify 认识,鉴定,确定generality 一般(性),一般原则,普遍(性),通则nomenclature 命名routinely 常规,惯例counterpart 对手modest 小的reversible 可逆的traced out 探寻踪(轨)迹primitive 原始的,早期的,开始的,基本的,简单的visualize 目测,观察,设想relativistic 相对论的aligned 排列好的distinction (差)区别,特性tetrahedrally 四面体的octahedrally 八面体的inventory 清单,目录cancellation 抵(取)消traverse 在?? 上来回移动,沿? 来回移动flunctuate 波动,涨落,起伏,动摇不定ingot 铸模,铸块,锭fidelity 保真consistent with 与一致emphasis 强调,重点,重要性axially 轴向weighted average 加权平均elementary 基本的reverse 相反的rigorous 严格的,严密的,精确的bound 限度take for granted 被忽略(视)communicate 传达,传递deflect 偏转unless otherwise state 除非另外说明appreciation 正确评价,鉴别substantial 多的,大的,大量的offset 弥补,抵消,偏移assembly 装配,组装,总成Samarium 钐Alnico 磁钢simultaneously 同时发生的product? (乘)积solenoid 螺线管deflection 偏转interchangeably 可交(互)换的,可代替的gem 宝石dodecahedral 十二面体的waveguide 波导hexagonal 六方晶系的strontium 锶fortuitous 偶然的,幸运的perovskite 钙钛矿availability 利用(或获得)的可能性levitation 悬浮application 应用mechanical property 力学性能constitute 组成stress 压力strain 应变hardness 硬度impact strength 冲击抗强度fracture toughness 断裂韧度fatigue 疲劳creep 蠕变/ 徐变ferrite 铁素体matrix 基体ductility 延展性corrosion resistance 抗腐蚀性alloying element 合金元素cast iron 铸铁brittleness 脆性spheroidal 类似球体的toughness 韧性tensile strength 抗张强度embrittling 使脆化metastable 亚稳的wear resistance 耐磨性abrasion 磨损superconducting 超导(电)的superplastic 超塑性的superalloys 超耐热合金light-sensitive 感光的elastic limit 弹性极限yield strength 屈服强度crack 裂缝crystalline 晶体elastomer 弹性体metal 金属microcrystalline 微晶的ceramic 陶瓷microstructure 微观结构chemical 化学的nano ceramic 纳米陶瓷chemical bond 化学键non-crystal 非晶体composite 复合材料composition 组成conductivity 导电性cost 成本physical property 物理性能defect structure 缺陷electron microscopy 电子显微镜engineering 工程semiconductor 半导体expansion 膨胀smart Ceramics 机敏陶瓷experiment 实验sintering 烧结fiber 纤维structure 结构synthesis 合成glass 玻璃technology 工艺temperature 温度thermal shock 热冲击inorganic 无机的thermal stability 热稳定性admixture 外加剂hydration 水化clay 粘土refractory 耐火材料reinforcement 增强sample 样品crystalline modification 晶型转化drying 干燥sand 砂polymer 聚合物thermosetting 热硬化性的thermoplastic 热塑性的organic 有机的concrete 混凝土cement 水泥brick 砖masonry 石质的calcium silicate 硅酸盐aggregate 骨料insulation 绝热coarse 粗的characterization 特性oxide 氧化物coal 煤powder 粉末process 加工furnace 熔炉formation 成型test 测试water 水alloy 合金fabrication 制备resin 树脂solder 焊接, 焊料crystal boundary 晶界torsion 扭转treatment 处理。
结构化学 英语
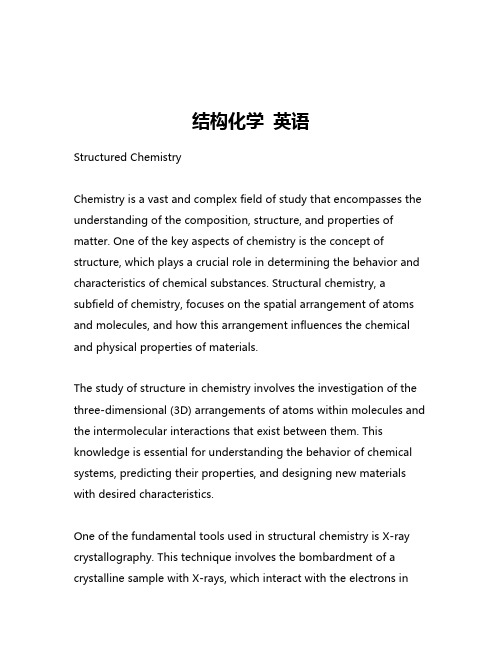
结构化学英语Structured ChemistryChemistry is a vast and complex field of study that encompasses the understanding of the composition, structure, and properties of matter. One of the key aspects of chemistry is the concept of structure, which plays a crucial role in determining the behavior and characteristics of chemical substances. Structural chemistry, a subfield of chemistry, focuses on the spatial arrangement of atoms and molecules, and how this arrangement influences the chemical and physical properties of materials.The study of structure in chemistry involves the investigation of the three-dimensional (3D) arrangements of atoms within molecules and the intermolecular interactions that exist between them. This knowledge is essential for understanding the behavior of chemical systems, predicting their properties, and designing new materials with desired characteristics.One of the fundamental tools used in structural chemistry is X-ray crystallography. This technique involves the bombardment of a crystalline sample with X-rays, which interact with the electrons inthe atoms of the crystal. The resulting diffraction pattern can be analyzed to determine the precise arrangement of atoms within the crystal structure. This information is crucial for understanding the properties of solid-state materials, such as metals, minerals, and ceramics.Another important technique in structural chemistry is nuclear magnetic resonance (NMR) spectroscopy. This method utilizes the magnetic properties of atomic nuclei to provide information about the chemical environment and connectivity of atoms within a molecule. NMR spectroscopy is widely used in the identification and characterization of organic compounds, as well as in the study of biomolecules, such as proteins and nucleic acids.In addition to these experimental techniques, computational methods have also become increasingly important in the field of structural chemistry. Quantum mechanical calculations, such as density functional theory (DFT), allow researchers to model the behavior of atoms and molecules at the quantum level, providing insights into their electronic structure and chemical reactivity.One of the key applications of structural chemistry is in the design and development of new materials. By understanding the relationship between the structure of a material and its properties, chemists can engineer substances with specific characteristics, suchas high strength, enhanced thermal stability, or improved electrical conductivity. This knowledge is particularly valuable in fields like materials science, nanotechnology, and catalysis.Another important aspect of structural chemistry is its role in the study of biological systems. The structures of proteins, nucleic acids, and other biomolecules are crucial for understanding their functions and interactions within living organisms. This knowledge is essential for the development of new drugs and the understanding of disease processes.In conclusion, the field of structural chemistry is a fundamental and multifaceted discipline that underpins our understanding of the physical and chemical properties of matter. Through the use of advanced experimental and computational techniques, structural chemists continue to unravel the mysteries of the molecular world, paving the way for new discoveries and innovations that have the potential to transform our lives.。
锆钛矿英语词汇大全
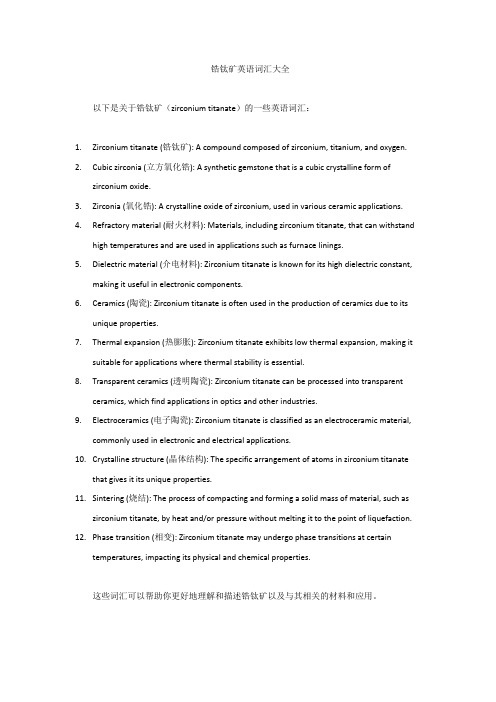
锆钛矿英语词汇大全以下是关于锆钛矿(zirconium titanate)的一些英语词汇:1.Zirconium titanate (锆钛矿): A compound composed of zirconium, titanium, and oxygen.2.Cubic zirconia (立方氧化锆): A synthetic gemstone that is a cubic crystalline form ofzirconium oxide.3.Zirconia (氧化锆): A crystalline oxide of zirconium, used in various ceramic applications.4.Refractory material (耐火材料): Materials, including zirconium titanate, that can withstandhigh temperatures and are used in applications such as furnace linings.5.Dielectric material (介电材料): Zirconium titanate is known for its high dielectric constant,making it useful in electronic components.6.Ceramics (陶瓷): Zirconium titanate is often used in the production of ceramics due to itsunique properties.7.Thermal expansion (热膨胀): Zirconium titanate exhibits low thermal expansion, making itsuitable for applications where thermal stability is essential.8.Transparent ceramics (透明陶瓷): Zirconium titanate can be processed into transparentceramics, which find applications in optics and other industries.9.Electroceramics (电子陶瓷): Zirconium titanate is classified as an electroceramic material,commonly used in electronic and electrical applications.10.Crystalline structure (晶体结构): The specific arrangement of atoms in zirconium titanatethat gives it its unique properties.11.Sintering (烧结): The process of compacting and forming a solid mass of material, such aszirconium titanate, by heat and/or pressure without melting it to the point of liquefaction.12.Phase transition (相变): Zirconium titanate may undergo phase transitions at certaintemperatures, impacting its physical and chemical properties.这些词汇可以帮助你更好地理解和描述锆钛矿以及与其相关的材料和应用。
材料的结构和组成

(b) The fractions of proeutectoid ferrite and pearlite are determined
(c) sum of these two ferrite fractions
4. 钢和铸铁 teel and Cast Iron
(1) 钢——碳含量低于1.2%(重量)的铁碳合金。 在金属中,普通碳钢、低合金钢<5%镍、铬。 (2) 铸铁——碳含量高于2% (重量)的铁碳合金。有 灰色铸铁、球墨铸铁和可锻铸铁。 灰铸铁, 2.5—3.5 %, 钢 +片状石墨
5个单相区,
7个两相区
三条特征线
三条水平线,
2. 典型铁碳合金结晶过程分析
(1)共析钢 eutectoid steel 0.77%C
(2)亚共析钢 hypoeutectoid steel 0.0218~0.77%C
(3)过共析钢 hypereutectoid steel 0.77~2.11%C
由电子。 Metallic bonding: ion cores electron cloud(sea of electrons)
2. 金属晶体结构 Clystal Structures of Metals (1)随温度变化 (2)室温下有三种:
体心立方结构 面心立方 密堆六方 较高强度、硬度和熔 具有良好塑性 强度低,塑 点但塑性和韧性差 和韧性 性和韧性差 Li, Na, K(S1) Au, Ag, Cu(d10s1) Mg, Ti, Zr(d2s2) Ni,Pd, Pt (d8s2) Cr, Mo, W (d5s1) 晶胞内 原子数 2 4 6 点阵常数 4R/3 4R/2 ——
(4)共晶白口铸铁 4.3%C
(5)亚共晶白口铸铁
晶体结构

晶体结构(structure of crystal)原子质量单位Atomic mass unit (amu)原子量Atomic weight键能Bonding energy共价键Covalent bond电子构型electronic configuration正电的Electropositive氢键Hydrogen bond同位素Isotope摩尔Mole泡利不相容原理Pauli exclusion principle 原子atom分子量molecule weight量子数quantum number范德华键van der waals bond点群point group各向异性anisotropy体心立方结构body-centered cubic (BCC) 布拉格定律bragg’s law晶体结构crystal structure晶体的crystalline中子衍射neutron diffraction晶界grain boundary鲍林规则Pauling’s rulesCsCl型结构Caesium Chloride structure 纤锌矿型结构Wurtzite structure萤石型结构Fluorite structure尖晶石型结构Spinel-type structure岛状结构Island structure层状结构Layer structure滑石talc高岭石kaolinite长石feldspar各向同性的isotropic晶格lattice密勒指数miller indices多晶的polycrystalline原子数Atomic number波尔原子模型Bohr atomic model库仑力Coulombic force分子的构型molecular configuration负电的Electronegative基态Ground state离子键Ionic bond金属键Metallic bond分子Molecule元素周期表Periodic table极性分子Polar molecule价电子valence electron电子轨道electron orbitals对称要素symmetry elements原子堆积因数atomic packing factor(APF) 面心立方结构face-centered cubic (FCC) 配位数coordination number晶系crystal system衍射diffraction电子衍射electron diffraction六方密堆积hexagonal close-packed (HCP) NaCl型结构NaCl-type structure闪锌矿型结构Blende-type structure金红石型结构Rutile structure钙钛矿型结构Perovskite-type structure硅酸盐结构Structure of silicates链状结构Chain structure架状结构Framework structure叶蜡石pyrophyllite美橄榄石forsterite各向异性的anisotropy晶格参数lattice parameters非结晶的noncrystalline多晶形polymorphism单晶single crystal电位electron states电子electrons金属键metallic bonding极性分子polar molecules衍射角diffraction angle粒度,晶粒大小grain size显微照相photomicrograph透射电子显微镜transmission electron microscope (TEM) 四方的tetragonal配位数coordination number晶胞unit cell(化合)价valence共价键covalent bonding离子键Ionic bonding原子面密度atomic planar density显微结构microstructure扫描电子显微镜scanning electron microscope (SEM) 重量百分数weight percent单斜的monoclinic晶体结构缺陷(defect of crystal structure)缺陷defect, imperfection线缺陷line defect, dislocation体缺陷volume defect位错线dislocation line螺位错screw dislocation晶界grain boundaries小角度晶界tilt boundary,位错阵列dislocation array位错轴dislocation axis位错爬移dislocation climb位错滑移dislocation slip位错裂纹dislocation crack位错密度dislocation density间隙原子interstitial atom间隙位置interstitial sites弗伦克尔缺陷Frenkel disorder主晶相the host lattice缔合中心Associated Centers.电子空穴Electron Holes克罗各-明克符号Kroger Vink notation 固溶体solid solution化合物compound置换固溶体substitutional solid solution 不混溶固溶体immiscible solid solution 有序固溶体ordered solid solution固溶强化solid solution strengthening 点缺陷point defect面缺陷interface defect位错排列dislocation arrangement刃位错edge dislocation混合位错mixed dislocation大角度晶界high-angle grain boundaries 孪晶界twin boundaries位错气团dislocation atmosphere位错胞dislocation cell位错聚结dislocation coalescence位错核心能量dislocation core energy 位错阻尼dislocation damping原子错位substitution of a wrong atom晶格空位vacant lattice sites杂质impurities肖脱基缺陷Schottky disorder错位原子misplaced atoms自由电子Free Electrons伯格斯矢量Burgers中性原子neutral atom固溶度solid solubility间隙固溶体interstitial solid solution金属间化合物intermetallics转熔型固溶体peritectic solid solution无序固溶体disordered solid solution取代型固溶体Substitutional solid solutions过饱和固溶体supersaturated solid solution非化学计量化合物Nonstoichiometric compound 表面结构与性质(structure and property of surface) 表面surface同相界面homophase boundary晶界grain boundary小角度晶界low angle grain boundary共格孪晶界coherent twin boundary错配度mismatch重构reconstuction表面能surface energy扭转晶界twist grain boundary共格界面coherent boundary非共格界面noncoherent boundary应变能strain energy惯习面habit plane界面interface异相界面heterophase boundary表面能surface energy大角度晶界high angle grain boundary晶界迁移grain boundary migration驰豫relaxation表面吸附surface adsorption倾转晶界titlt grain boundary倒易密度reciprocal density半共格界面semi-coherent boundary界面能interfacial free energy晶体学取向关系crystallographic orientation非晶态结构与性质(structure and property of uncrystalline) 熔体结构structure of melt玻璃态vitreous state粘度viscosity介稳态过渡相metastable phase淬火quenching玻璃分相phase separation in glasses 过冷液体supercooling melt软化温度softening temperature表面张力Surface tension组织constitution退火的softened体积收缩volume shrinkage扩散(diffusion)活化能activation energy浓度梯度concentration gradient菲克第二定律Fick’s second law稳态扩散steady state diffusion扩散系数diffusion coefficient填隙机制interstitalcy mechanism短路扩散short-circuit diffusion下坡扩散Downhill diffusion扩散通量diffusion flux菲克第一定律Fick’s first law相关因子correlation factor非稳态扩散nonsteady-state diffusion 跳动几率jump frequency晶界扩散grain boundary diffusion上坡扩散uphill diffusion互扩散系数Mutual diffusion渗碳剂carburizing浓度分布曲线concentration profile 驱动力driving force自扩散self-diffusion空位扩散vacancy diffusion扩散方程diffusion equation扩散特性diffusion property达肯方程Dark equation本征热缺陷Intrinsic thermal defect 离子电导率Ion-conductivity浓度梯度concentration gradient扩散流量diffusion flux间隙扩散interstitial diffusion表面扩散surface diffusion扩散偶diffusion couple扩散机理diffusion mechanism无规行走Random walk柯肯达尔效应Kirkendall equation本征扩散系数Intrinsic diffusion coefficient 空位机制Vacancy concentration腐蚀与氧化(corroding and oxidation)氧化反应Oxidation reaction还原反应Reduction reaction价电子Valence electron腐蚀介质Corroding solution电动势Electric potential推动力The driving force腐蚀系统Corroding system腐蚀速度Corrosion penetration rate电流密度Current density电化学反应Electrochemical reaction极化作用Polarization过电位The over voltage浓差极化Concentration polarization电化学极化Activation polarization极化曲线Polarization curve缓蚀剂Inhibitor原电池galvanic cell电偶腐蚀galvanic corrosion电位序galvanic series应力腐蚀Stress corrosion冲蚀Erosion-corrosion腐蚀短裂Corrosion cracking防腐剂Corrosion remover腐蚀电极Corrosion target隙间腐蚀Crevice corrosion均匀腐蚀Uniform attack晶间腐蚀Intergranular corrosion焊缝破坏Weld decay选择性析出Selective leaching氢脆损坏Hydrogen embitterment阴极保护Catholic protection穿晶断裂Intergranular fracture固相反应和烧结(solid state reaction and sintering) 固相反应solid state reaction烧成fire再结晶Recrystallization成核nucleation子晶,雏晶matted crystal异质核化heterogeneous nucleation铁碳合金iron-carbon alloy铁素体ferrite共晶反应eutectic reaction烧结sintering合金alloy二次再结晶Secondary recrystallization结晶crystallization耔晶取向seed orientation均匀化热处理homogenization heat treatment渗碳体cementite奥氏体austenite固溶处理solution heat treatment相变(phase transformation)过冷supercooling晶核nucleus形核功nucleation energy均匀形核homogeneous nucleation形核率nucleation rate热力学函数thermodynamics function临界晶核critical nucleus枝晶偏析dendritic segregation平衡分配系数equilibrium distribution coefficient成分过冷constitutional supercooling共晶组织eutectic structure伪共晶pseudoeutectic表面等轴晶区chill zone中心等轴晶区equiaxed crystal zone急冷技术splatcooling单晶提拉法Czochralski method位错形核dislocation nucleation斯宾那多分解spinodal decomposition马氏体相变martensite phase transformation 成核机理nucleation mechanism过冷度degree of supercooling形核nucleation晶体长大crystal growth非均匀形核heterogeneous nucleation长大速率growth rate临界晶核半径critical nucleus radius局部平衡localized equilibrium有效分配系数effective distribution coefficient 引领(领先)相leading phase层状共晶体lamellar eutectic离异共晶divorsed eutectic柱状晶区columnar zone定向凝固unidirectional solidification区域提纯zone refining晶界形核boundary nucleation晶核长大nuclei growth有序无序转变disordered-order transition马氏体martensite成核势垒nucleation barrier相平衡与相图(Phase equilibrium and Phase diagrams)相图phase diagrams组分component相律Phase rule浓度三角形Concentration triangle成分composition相平衡phase equilibrium热力学thermodynamics吉布斯相律Gibbs phase rule吉布斯自由能Gibbs free energy吉布斯熵Gibbs entropy热力学函数thermodynamics function过冷supercooling杠杆定律lever rule相界线phase boundary line共轭线conjugate lines相界反应phase boundary reaction相组成phase composition金相相组织phase constentuent相衬显微镜phase contrast microscope 相分布phase distribution相平衡图phase equilibrium diagram 相分离phase segregation相phase组元compoonent投影图Projection drawing冷却曲线Cooling curve自由度freedom化学势chemical potential相律phase rule自由能free energy吉布斯混合能Gibbs energy of mixing 吉布斯函数Gibbs function热分析thermal analysis过冷度degree of supercooling相界phase boundary相界交联phase boundary crosslinking相界有限交联phase boundary crosslinking 相变phase change共格相phase-coherent相衬phase contrast相衬显微术phase contrast microscopy相平衡常数phase equilibrium constant相变滞后phase transition lag相序phase order相稳定性phase stability相稳定区phase stabile range相变压力phase transition pressure同素异晶转变allotropic transformation显微结构microstructures不混溶性immiscibility相态phase state相变温度phase transition temperature同质多晶转变polymorphic transformation 相平衡条件phase equilibrium conditions 低共熔体eutectoid。
二维晶体材料的结构缺陷
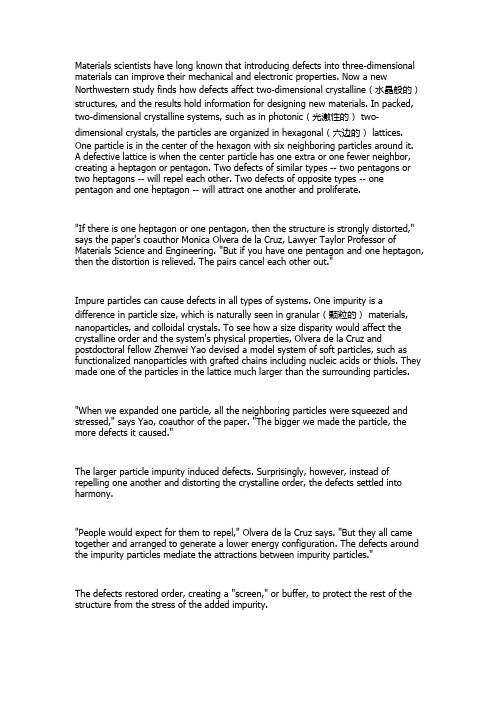
Materials scientists have long known that introducing defects into three-dimensional materials can improve their mechanical and electronic properties. Now a new Northwestern study finds how defects affect two-dimensional crystalline(水晶般的)structures, and the results hold information for designing new materials. In packed, two-dimensional crystalline systems, such as in photonic(光激性的) two-dimensional crystals, the particles are organized in hexagonal(六边的) lattices. One particle is in the center of the hexagon with six neighboring particles around it.A defective lattice is when the center particle has one extra or one fewer neighbor, creating a heptagon or pentagon. Two defects of similar types -- two pentagons or two heptagons -- will repel each other. Two defects of opposite types -- one pentagon and one heptagon -- will attract one another and proliferate."If there is one heptagon or one pentagon, then the structure is strongly distorted," says the paper's coauthor Monica Olvera de la Cruz, Lawyer Taylor Professor of Materials Science and Engineering. "But if you have one pentagon and one heptagon, then the distortion is relieved. The pairs cancel each other out."Impure particles can cause defects in all types of systems. One impurity is a difference in particle size, which is naturally seen in granular(颗粒的) materials, nanoparticles, and colloidal crystals. To see how a size disparity would affect the crystalline order and the system's physical properties, Olvera de la Cruz and postdoctoral fellow Zhenwei Yao devised a model system of soft particles, such as functionalized nanoparticles with grafted chains including nucleic acids or thiols. They made one of the particles in the lattice much larger than the surrounding particles."When we expanded one particle, all the neighboring particles were squeezed and stressed," says Yao, coauthor of the paper. "The bigger we made the particle, the more defects it caused."The larger particle impurity induced defects. Surprisingly, however, instead of repelling one another and distorting the crystalline order, the defects settled into harmony."People would expect for them to repel," Olvera de la Cruz says. "But they all came together and arranged to generate a lower energy configuration. The defects around the impurity particles mediate the attractions between impurity particles."The defects restored order, creating a "screen," or buffer, to protect the rest of the structure from the stress of the added impurity.This finding could lead to new ways of engineering materials, supporting the Materials Genome Initiative. Creating materials with new properties by adding impurities can be tricky. If the impurities cause defects that induce attractionsbetween impurity particles, then they might create regions where impurities aggregate. "That generates an interface of two materials that can be very damaging," Olvera de la Cruz says. "The impurities have to be very well controlled."By changing the size of particles, materials researchers may be able to engineer defects in a convenient and precise manner.更多英语学习:企业英语/。
武汉理工大学研究生--材料科技英语-课文翻译
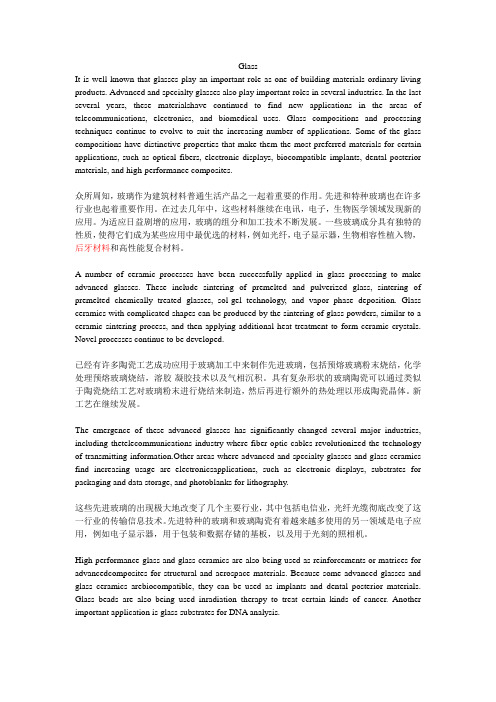
GlassIt is well known that glasses play an important role as one of building materials ordinary living products. Advanced and specialty glasses also play important roles in several industries. In the last several years, these materialshave continued to find new applications in the areas of telecommunications, electronics, and biomedical uses. Glass compositions and processing techniques continue to evolve to suit the increasing number of applications. Some of the glass compositions have distinctive properties that make them the most preferred materials for certain applications, such as optical fibers, electronic displays, biocompatible implants, dental posterior materials, and high-performance composites.众所周知,玻璃作为建筑材料普通生活产品之一起着重要的作用。
先进和特种玻璃也在许多行业也起着重要作用。
在过去几年中,这些材料继续在电讯,电子,生物医学领域发现新的应用。
为适应日益剧增的应用,玻璃的组分和加工技术不断发展。
【人物与科研】华中科技大学夏宝玉...

【人物与科研】华中科技大学夏宝玉...导语金属有机框架材料(MOFs)是由有机配体和金属离子或团簇配位形成的配合物。
由于其可控的形貌、高比表面积和多孔结构而被广泛应用于储能领域。
MOFs材料中的金属组分对电极材料的性能有很大影响,通过金属离子的活化可以有效提升材料性能,然而这种活化往往伴随着结构的严重破坏而没法最大限度提升材料电化学性能。
近日,华中科技大学夏宝玉课题组通过一种氧化还原的方法调控双金属Co-Ni MOF中的金属组分得到稳定低价的混合价态的Co-Ni MOF 衍生物,得到的材料具有优异的电化学性能。
相关成果在线发表于Adv. Mater.(DOI: 10.1002/adma.201905744)。
夏宝玉教授课题组主要从事结构功能材料及其在能量转换与存储等领域的研究工作。
团队围绕新材料在新能源技术中的服役和失效问题,以高活性长寿命低成本电极材料为导向,通过探究材料腐蚀现象和规律,利用传统腐蚀科学与技术开发新型、稳定的材料与器件,使其达到高水平长寿命服役的目的,实现传统腐蚀学科与新能源领域的深度交叉融合。
夏宝玉,教授/博士生导师,2010年毕业于上海交通大学,获得博士学位。
2011-2016年在新加坡南洋理工大学从事博士后研究作,2016年入职华中科技大学,入选中组部第十二批“计划”。
近年来在包括Science, Nature Energy, J. Am. Chem. Soc., Angew. Chem. Int. Ed., Adv. Mater.等国际期刊发表学术论文70余篇(包括1篇ESI热点论文和21篇ESI高被引论文),发表的研究工作被国内外同行引用8000余次,参与3本专著编写(三章),申请专利6项(已授权3项),H指数29,研究成果被Chemistry Views,Materials Views 等媒体报道。
夏宝玉教授入选2018年、2019年科睿唯安全球高被引科学家和2019年Journal of Materials Chemistry A Emerging Investigators。
- 1、下载文档前请自行甄别文档内容的完整性,平台不提供额外的编辑、内容补充、找答案等附加服务。
- 2、"仅部分预览"的文档,不可在线预览部分如存在完整性等问题,可反馈申请退款(可完整预览的文档不适用该条件!)。
- 3、如文档侵犯您的权益,请联系客服反馈,我们会尽快为您处理(人工客服工作时间:9:00-18:30)。
Digest Journal of Nanomaterials and Biostructures Vol. 1, No. 3, September 2006, p. 71 – 79 CRYSTALLINE AND ELECTRONIC STRUCTURE OF GOLD NANOCLUSTERS DETERMINED BY EXAFS, XRD AND XPS METHODSAldea∗a, P. Marginean a, V. Rednic a, S. Pintea a , B. Barz∗∗b A. Gluhoi c,N.B. E. Nieuwenhuys c, Xie Yaning d, F. Aaldea e,M. Neumann fa National Institute for Research and Development of Isotopic and Molecular Technologies, Romaniab University of Missouri-Columbia, 223 Phys Building MO 65211c Leiden Institute of Chemistry, Leiden University, The Netherlandsd Beijing Synchrotron Radiation Facilities of Beijing Electron Positron Collider,Coordination Laboratory of National Center for Nanoscience and Nanotechnology, Beijing, People's Republic of Chinae University of Agriculture Sciences and Veterinary Medicine, Math. Department. Cluj-RomaniaNapoca,fUniversity of Osnabrück, Fachbereich Physik, 49069 Osnabrück, GermanyGold particles nanoclusters as supported catalysts were analyzed by extended X-rayabsorption fine structure, X-ray diffraction and X-ray photoelectron spectroscopy in order todetermine their local, global and electronic structure. The present study points out the strongdeformation of the local structure of the active metal due to its interaction with oxidesupports as well as a possible oxidation state of the gold component. The average particlesize and the microstrain parameters of the supported Au nanoclusters were determined by X-ray diffraction method, based on the Fourier transform of the experimental X-ray lineprofiles. The global structure is obtained with a fitting method using of the generalizedFermi function facilities for the approximation technique. The strong interaction between themetal nanoclusters and oxide supports induces a great change in the local and globalmicrostructures of the catalysts, modifying at the same time the chemisorptions and catalyticprocesses that occur at the Au-support interface. The following catalyst samples wereinvestigated: Au/ZnO/MgO/Al2O3,Au/CuO/Al2O3 and Au/Li2O/CeO2/Al2O3. The XPStechnique was used for determining the electronic states of gold, gold oxide and metal oxidesin the oxide supports.Keywords: Supported gold catalysts, X-ray diffraction, X-ray absorption spectroscopy,X-ray photoelectron spectroscopy, Metal support interaction, Local and global structure.Introduction1.A short theoretical background of the extended X-ray absorption fine structure (EXAFS) and the X-ray diffraction (XRD) analyses, including the main mathematical properties of the generalized Fermi function (GFF), used for approximation and deconvolution of the experimental X-ray line profiles (XRLP) are presented in sections one and three of the paper [1-3]. The fluorescence EXAFS measurements on L III gold edge were carried out in 4W1B beam lines operating at 30-50∗ Corresponding author: naldea@s3.itim-cj.ro∗∗ On leave from National Institute for R & D of Isotopic and Molecular Technologies, Romania72mA and 2.2 GeV with an energy resolution of 0.5-3 eV at 10 KeV, at room temperature [4]. The X-ray (111), (200), (220) and (311) diffraction data for supported gold catalysts were collected using a Rigaku rotating anode setup, in Bragg-Brentano geometry with Ni filtered Cu K α radiation, λ=1.5406 Å at room temperature. A NaI (Tl) detector was used and the signals were amplified and fed to a single channel analyzer (ORTEC 850) read out by a computer.The XPS spectra were recorded using a PHI 5600ci ESCA spectrometer with monochromatic Al K α X-ray radiation (1486.6 eV) at room temperature. The pressure in the ultra-high vacuum chamber was within the 10-10 mbar range during the measurements. The neutralizer was used in every case due to a charge effect which occurs for nonconducting samples. The binding energy was determined by reference to the C 1s line at 284.8 eV.Supported gold catalysts were prepared by homogeneous deposition-precipitation with urea on support of MO x /Al 2O 3 (M: Cu, Ce, Mg, Li in different combinations). The gold precursor was HAuCl 4·3H 2O, Aldrich, 99.999% purity [5]. The theoretical gold content for the catalysts was 5 wt %. The catalysts were calcined at 3000 C before the measurements.2. EXAFS analysisThe interference between the outgoing and backscattered electron waves has the effect of modulating the X-ray absorption coefficient. The EXAFS function χ(k ) is defined in terms of the atomic absorption coefficient by,)()()()(00k k k x µµµχ−=(1) The theoretical EXAFS signal is defined by the relation∑+=jj j j k kr k A k )](2sin[)()(δχ, (2) where )2exp()](/2exp[),,()(222j j j j j k k r r k F kr N k A σλπ−−⎟⎟⎠⎞⎜⎜⎝⎛=, r j - the radial distance, N j – the number of atoms in the j th shell, δj (k) - the phase shift function, σj - the root mean square deviation of the distance about r j , F(k, r, π) - the backscattering and λj (k) - the mean free path function for inelastic scattering. The single shell contribution may be isolated by Fourier transform technique∫−=Φmaxmin )()2exp()()2/1()(2/1k k n dk k WF ikr k k r χπ, (3)where WF(k) is the window function used for the minimization of the spurious errors. The inverse Fourier transform of the radial structure function is obtained for any coordination shell with:.)2exp()()()/1()/2()(212/1dr ikr r k WF k k jj R R n n j ∫Φ=πχ(4)The structural parameters for each coordination shell are determined by fitting the experimental spectrum with the theoretical function [1,2].3. X-ray diffraction analysisThe global structure is obtained with a new fitting method based on the GFF facilities for approximation and the Fourier transform of the experimental XRLP and the instrumental function. The GFF is a simple function with a minimal number of parameters, suitable for XRLP global approximation, based on minimization methods [1] and it is defined by the relation)c s (b )c s (a e e A)s (h −−−+= (5)73where A, a, b, c are unknown parameters and s = 2 sin(θ) / λ. The values A and c describe the amplitude and the position of the XRLP while a and b control its shape.The integral width of the GFF distribution and the module of the Fourier transform for the true sample were determined by the deconvolution technique and they have the following forms()⎟⎠⎞⎜⎝⎛+−ππ=δ+b a b a 2cos b a )b ,a ()b a /(1b a (6) L sinh cos L sinh cos A A )L (F 2222h g g h δ+γβ+αρρ= (7) where 2b a +=ρ, 2b a q −=, g g q ρπα2=, g ρπβ2=, hh q ρπγ2= and h ρπδ2= The true sample XRLP and its integral width are given by the relations:***cosh 2cos cosh 2cos 2)(s s A A s f h gh h gh g g h ρρπρρρπρπρ+= (8) ⎟⎟⎠⎞⎜⎜⎝⎛+=1cos 2cos 2),(g h gh h g h ρπρρπρρπρρδ (9) where the indices h, g refer to the experimental and the instrumental profiles. Based on Warren and Averbach theory, the general form of the Fourier transform of the true sample for cubic lattices is obtained from the general relation [3],⎟⎟⎟⎠⎞⎜⎜⎜⎝⎛επ+−=220hkl 2L 2eff a L h 2)hkl (D |L |e )L (F (10)where D eff (hkl) is the effective crystallite size, <ε2>hkl is the microstrain of the lattice and 22220l k h h ++=. The data processing of the XRLP presented in the third section is based on the GFF approximation and on its remarkable properties. The effective crystallite size, D eff (hkl) and the microstrain of the lattice <ε2>hkl were calculated using Eqs. (5-10). Our computer package program SIZE, written in Maple language, has implemented all the procedures for the global structure determination of the nanostrucured materials by data processing of the XRLP.74Fig. 1. The normalized absorption coefficients of Au L III edge for the investigated samples.Fig. 2. The relative intensity of (111), (200), (220) and (311) experimental XRPL for Au foil as the instrumental function.Fig. 3. The relative intensity of (111), (200), (220) and (311) experimental XRPL for (2), (3)and (10) supported gold catalysts samples.75 analysisXPS4.X-ray photoelectron spectroscopy is widely used in catalysis because it allows determination of the chemical state of the elements of solid surface such as oxidation number and the electronic interactions with neighboring elements from the first coordination shells. In the case of reducible metals the XPS information are very complementary to those of the temperature programmed reduction (TPR). SimPeak computer package program was used for data analysis of the XPS spectra. They are global approximated by the asymmetric Donjac-Sunjic distribution using Levenberg Marquardt algorithm. For background correction we used Tougaard algorithm based on mathematical description of the electron transport.discussion5.andResultsresultsEXAFS5.1.In Figs. 1-3 the numbers (0), (2), (3) and (10) represent the spectra of gold foil used as instrumental correction in the XRD analysis for Au/ ZnO/MgO/Al2O3, Au/ CuO/Al2O3 and Au(Au2O3)/ Li2O/CeO2/Al2O3. The EXAFS experimental spectra are given in Fig. 1. The extraction of EXAFS signal is based on the determination of the threshold energy for Au L III edge followed by pre-edge and after-edge background removal with base line fitting with different modeling functions and by µ0(k) and µ(k) evaluation with 3rd order bell spline functions. In according with Eq. (1) the EXAFS signals were performed in the range 37.5-140 nm-1. In order to obtain the distribution of the atomic distances we calculated the radial structure function using Eq. (3) [1-2, 6]. The mean Au-Au distances of the first and the second coordination shell for standard sample, at room temperature have values close to R1=0.287 and R2=0.407 nm. Based on the Fourier transform of k3χ(k)WF(k), performed in the range 0.02-0.5 nm, we obtained the magnitude of the atomic radial distribution |Φ(r)| for investigated samples as well as for standard Au foil. In order to avoid spurious errors due to limited interval in the wave vector space we have taken into consideration the Kaiser window function [7-8]. The Fourier transform magnitude is diminished as a result of the reduced average co-ordination number and the peaks in |Φ(r)| are shifted from the true distance due to the phase shift function that is included in the EXAFS signal. We proceeded by taking the inverse Fourier transform given by Eq (4) of the first neighboring peak and then extracting the amplitude function A j(k) and the phase shift function δ(k) in according with Eq. (2). By Lavenberg-Marquard fit of Eq. (2) and experimental contribution given by Eq.(4) for each coordination shell, we then evaluated the interatomic distances, number of neighbours and edge position. Table I contains the best values of the local structure parameters of the investigated samples and the errors given for the best-fit parameters, ∆N and ∆R. The average inter-atomic distances obtained for the first coordination shell have practically the same values as those of the standard sample. There was a difference of approximately 27% between the number of atoms of investigated catalysts and the standard sample (N1=12). We considered that this diminution of the number of atoms is due to a strong electron interaction between the Au active metal and oxide supports [9-11].Fig. 4. XPS survey spectrum of the Au(Au2O3)/Li2O/CeO2/Al203 sample.76Fig. 5. XPS spectrum of O 1s lines for Au(Au 2O 3)/Li 2O/CeO 2/Al 203 sample.Table 1. The local structural parameters of the investigated samples for the first coordination shell.Sample Atomic number N 1 ± ∆N 1 Shell radius [nm]R 1 ± ∆R 1Au Foil 12 0.287Au/ZnO/MgO/Al 2O 3 2.32±0.1 0.287±0.02Au/CuO/Al 2O 3 4.61±0.13 0.287±0.01Au/Li 2O/CeO 2/Al 2O 3 2.93±0.12 0.280±0.02Fig. 6. XPS spectrum of Ce 3d lines for Au(Au 2O 3)/Li 2O/CeO 2/Al 203 sample.5.2. XRD resultsGenerally, it is not easy to obtain accurate values of the crystallite size and the microstrain parameter without extreme care in the experimental measurements and analysis of XRD data. The Fourier analysis of XRLP depends strongly on the magnitude and nature of the errors propagated in the data analysis. Three systematic errors are treated in papers [12-13]: uncorrected constant background, truncation and the effect of sampling for the observed profile at a finite number of points that appear in the discrete Fourier analysis. In order to minimize the propagation of these77 systematic errors, a global approximation of the XRLP is adopted instead of the discrete mathematical analysis. Therefore, in this paper, the structural parameters obtained from the diffraction line broadening in the X-ray powder pattern were analytically calculated using the GFF facilities. The reason for choosing this method, as described in Section 3, was the simplicity and the mathematical elegance of the analytical form of the Fourier transform magnitude and the integral width of the true XRLP given by Eqs.(7,9). This method was chosen against a numerical fast Fourier transform (FFT) whose validity depends on the filtering technique adopted [14]. In this way the accuracy of the global nanostructural parameters are closely related to the accuracy of the Fourier transform magnitude of the true XRLP.Fig. 7. XPS spectrum of Au 4f lines for Au(Au2O3)/Li2O/CeO2/Al203 sample.The following profiles were processed: (111), (200), (220) and (311). Their experimental relative intensities with respect to the 2θ values and the Au foil spectrum as instrumental broadening effect are shown in Figs. 2-3. The background correction of the XRLP was done by polynomial procedures. The further steps in the analysis are the removal of the metallic oxides contribution from the XRLP, the doublet Rachinger correction [15] and the determination of the best parameters of the GFF distributions by nonlinear least squares fit. In order to determine the nanostructural parameters within Eq. (10) we computed the Fourier transforms of the true XRLP, and the integral width using Eqs. (7,9). In Section 4.2 we have shown that the coordination shells radii of the investigated samples have similar values as the Au foil. This important result is strongly correlated with the positions of (111), (200), (220) and (311) XRLP from the experimental spectra contained in Fig. 3. Therefore, these results explain the metal features of the bulk gold investigated clusters despite the strong deformation of the crystalline structure.Carbon oxide chemisorption, transmission electron microscopy, magnetization, electronic paramagnetic resonance and other methods could have been used to determine the grain size of the particles by taking into account a prior spherical form for the grains. By XRD method one can obtain the crystallite size that has different values for different crystallographic planes. There is a large difference between the grain size and the crystallite size due to the physical meaning of the two concepts. It is possible that the grains of the gold are built up of many gold crystallites. The global structural parameters obtained for the investigated samples are summarized in Table II. The microstrain parameter of the lattice can also be correlated with the effective crystallite size in the following way: the value of the effective crystallite size increases when the microstrain value decreases. On the other hand, in many cases, the nano-crystallite size determined by Scherrer equation is greater than the one obtained by Eq. (10) because it does not take into account the lattice strains. Therefore, the crystallite size values determined by classical Scherrer equation [16] for nanostructured materials as supported metal catalysts are less reliable than the results obtained from Fourier transform method described in Section 3.78Table 2. The global structure parameters of the investigated samples.Sample XRLPCrystallite size D eff [nm] Microstrain<ε2>hkl ×10-2Au/ZnO/MgO/Al 2O 3 (111) 1.6 2.06Au/CuO/Al 2O 3 (311) 5.8 0.40Au/Li 2O/CeO 2/Al 2O 3 (311) 6.0 0.385.3. XPS resultsThe survey photoemission spectrum of Au(Au 2O 3)/Li 2O/Ce 2O/Al 2O 3 catalyst with the identification of the main XPS core level lines are presented in Fig. 4. Besides the spectral features related to the constituent elements of the supported gold catalysts only a weak C 1s peak has been detected. The presence of the C element is due to hydrocarbons absorbed on the surface of the sample powder. Along with the main lines there are loss peaks which are weaker and broader than the photoelectron peaks and appear in the spectrum as though they are at higher binding energy. Their appearance is due to the small crystallite sizes of the investigated samples.In Fig. 5 is presented the O 1s line. It represents three contributions from oxide supports (Li 2O, CeO 2, Al 2O 3) and one that could be from Au oxide (Au 2O 3).Fig. 6 shows the contribution of the Ce 3d transitions containing six peaks. The peaks are spin-orbit split into a doublet, with each doublet showing further structure due to final state effects. In Fig. 7 is shown the XPS spectrum in the Au 4f region of the active metal. It can be seen that the background strongly increases at high energy. If an emitted electron has two or more inelastic collisions, it contributes to the general background of a spectrum, so the background commonly increases to the high binding energy side of any major photoelectron peak. This region shows two peaks, due to the Au 4f 7/2 and Au 4f 5/2 transitions. The 4f line is spllited due to the spin orbit coupling. These peaks are centered at about 85.5 eV and 89 eV. The value 85.5 eV of the Au 4f 7/2 is closely related to the 85.7 eV value, corresponding to Au oxide, than to Au in a metallic state (84 eV), which suggests that the gold is in an oxidized state. From previously information based on EXAFS and XRD measurements such as XRLP positions and the values of the first coordination shell, we evidenced only Au in a metal state. The oxidation state cannot be observed by EXAFS and XRD because these methods determine the crystalline structure in bulk gold crystallites while XPS gives information mainly from the surface of the investigated samples. This suggests that the Au oxidation state is present only on the surface of the crystallites [17-19].6. ConclusionsIn the present paper it has been shown that, in addition to EXAFS experiments with their specific advantages, XRD analysis can add more information for understanding bulk nanostructure of the gold nanoparticles and the XPS method gives us the electronic structure of gold nanoclusters and their multicomponents. The conclusions that can be drawn from these studies are:(i) The diminution of the number of atoms from the first and the second coordination shells of Au in the investigated samples points out the existence of an electronic interaction between the gold nanoparticles and the oxide supports;(ii) For XRLP analysis, a global approximation is applied rather than a numerical Fourier analysis. The former analysis is better than a numerical calculation because it minimizes the systematic errors that can appear in the traditional Fourier analysis.(iii) Our results showed that by using the GFF distribution we could successfully obtain reliable global nanostructural parameters.(iv) The XPS method provided us additional information related to the possible existence of the gold oxide on the surface of the gold crystallites for the last investigated sample.79 References[1] N. Aldea, Andreea Gluhoi, P. Marginean, C. Cosma, Xie Yaning, Spectrochim. Acta PartB 55, 997 (2000).[2] N. Aldea, Andreea Gluhoi, P. Marginean, C. Cosma, Xie Yaning, Hu Tiandou, Whongua Wu Baozhong Dong, Spectrochim. Acta B. Part B 57, 1453 (2002).[3] N. Aldea, B. Barz, T. D. Silipas, Florica Aldea and Zhonghua Wu, J. Optoelectron. Adv. Mater.7(6), 3093 (2005).[4] B.S.R.F. Activity Report 2003-2004, Beijing Electron Positron Collider National Laboratory.[5] R Grisel, Kees-Jan Weststrate, Andrea Gluhoi and B. E. Nieuwenhuys, Gold Bulletin35(2), 45 (2002).[5] F. W. Lytle, R. B. Greegor, E. C. Marques, D. R. Sandstrom, G. H. Via, J. H. Sinfelt, J.Catal. 95, 548 (1985).[6] San-Miguel, Physica B208/209, 177(1995).[7] N. Aldea, E. Indrea, Comput. Phys. Commun. 60, 145 (1990).[8] D. C. Koningsberger, B. C. Gates, Catal. Lett 14, 271 (1992).[9] C. H. Lin, S. H. Hsu, M.Y. Lee, S. D. Lin, J. Catal. 209, 62 (2002).[10] R. Zanella, S. Giorgio, C. H. Shin, C. R. Henry, C. Louis, J. Catal. 222, 357 (2004).[11] R. A. Young, R. J. Gerdes, A. J.Wilson, Acta Cryst. 22, 155 (1967).[12] N. Aldea, C. V. Tiusan, B. Barz, J. Optoelectron. Adv. Mater. 6(1) 225 (2004).[13] J. S. Walker, Fast Fourier Transform, Second Ed. CRC Boca Raton, New York, London,Tokyo, 1997.[14] N. Aldea, E. Indrea, Comput Phys. Commun. 60, 155 (1990).[15] H. P. Kug, L. E. Alexander, X-ray diffraction procedures for polycrystalline and amorphousmaterials, 2nd ed. John Wiley and Sons, New York 1974.[16] J. Guzman, B. C. Gates, J. Phys. Chem. B 106, 7659 (2002).[17] J. Hua, K. Wei, Q. Zheng, X. Lin, Appl. Catal. A 259, 121 (2003).[18] Z. Hao, A. Liduan, H. Wang, H.Tiandou, React. Kinet. Catal. Lett. 70, 153 (2000).。