Synthesis, Characterizations and Luminescent Properties
表面功能化CdS纳米微粒的制备

二 水 合 乙酸镉 , 基 乙醇 ( ) 硫脲 和 N, 巯 ME , N一 二 甲基 甲酰胺 ( DMF) 都是分 析 纯试 剂 , 用前 没 有 使
进 一步 纯化 。 一氨基 一1 1 一邻 菲 罗啉 ( h n 按 5 ,0 Ap e )
如D MF和二 甲亚砜( MS 中有很好的分散性 。 D O)
的C S纳米 微粒 , d 通过 I 测试 显示 , p e R A h n接枝 在C S纳 米微粒 的表 面 。 d 功能 化 后的C S纳 米微 粒 呈现 d
单分 散分 布 ,X RD谱 图表 明功 能化 的 AP e - C S微粒 和纯 的 C S纳米 微 粒相 比较 , 粒仍 然 是立 方 hn d d 微 晶的结 构且 粒径 没有发 生 改变仍 保持 3 n —5 m。 关键词 : h n C S纳 米微 粒 ; Ap e ; d 配体交 换 法 : 功能 化 的
Ma n 6 T—I 红 外光 谱仪 ; ime s g a5 0F R Se n D一 5 0 0 5X一射线 衍射 仪 C K(( 一1 5 1 7 ; u ( . 4 8 ) 1 2 原料Fra bibliotek和试 剂 .
次, 乙醚 洗涤 1 , 空 室温 干燥 , 后得 到 2 9 次 真 最 . g黄 色粉 末 。得到 的 Ap e -C S纳米微 粒 在极 性 溶剂 hn d
I mm u fu e c ntl b lng o a c rm a ke no l or s e a e i fc n e r r
He 2 a d o h rc l l rt r e s wih s mio — r n t e e l a a g t t e c n u
2 结果与 讨论
2T ea ̄ re h t ( e)
《近红外给体-受体AIE分子设计合成及光诊疗研究》范文
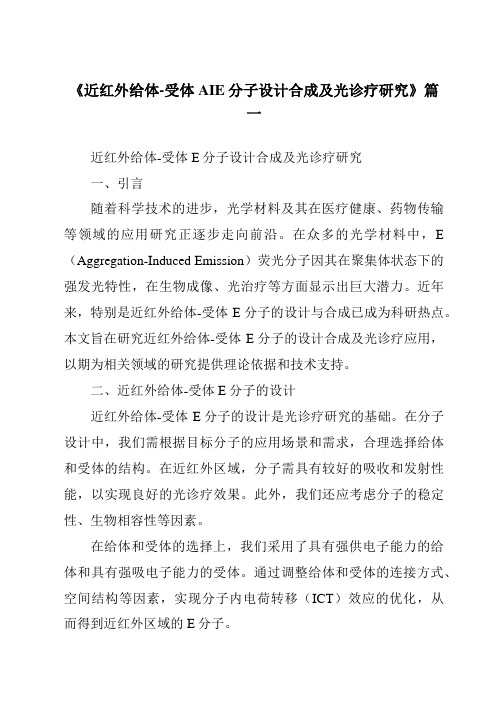
《近红外给体-受体AIE分子设计合成及光诊疗研究》篇一近红外给体-受体E分子设计合成及光诊疗研究一、引言随着科学技术的进步,光学材料及其在医疗健康、药物传输等领域的应用研究正逐步走向前沿。
在众多的光学材料中,E (Aggregation-Induced Emission)荧光分子因其在聚集体状态下的强发光特性,在生物成像、光治疗等方面显示出巨大潜力。
近年来,特别是近红外给体-受体E分子的设计与合成已成为科研热点。
本文旨在研究近红外给体-受体E分子的设计合成及光诊疗应用,以期为相关领域的研究提供理论依据和技术支持。
二、近红外给体-受体E分子的设计近红外给体-受体E分子的设计是光诊疗研究的基础。
在分子设计中,我们需根据目标分子的应用场景和需求,合理选择给体和受体的结构。
在近红外区域,分子需具有较好的吸收和发射性能,以实现良好的光诊疗效果。
此外,我们还应考虑分子的稳定性、生物相容性等因素。
在给体和受体的选择上,我们采用了具有强供电子能力的给体和具有强吸电子能力的受体。
通过调整给体和受体的连接方式、空间结构等因素,实现分子内电荷转移(ICT)效应的优化,从而得到近红外区域的E分子。
三、E分子的合成E分子的合成主要采用有机合成方法。
根据所设计的分子结构,我们通过多步反应,成功合成了近红外给体-受体E分子。
在合成过程中,我们严格控制反应条件,保证分子的纯度和质量。
四、E分子的光诊疗研究(一)光学性质研究我们对所合成的E分子进行了光学性质研究。
通过紫外-可见吸收光谱、荧光光谱等手段,我们发现该分子在近红外区域具有较好的吸收和发射性能。
此外,我们还研究了分子的光稳定性、量子产率等光学性质。
(二)生物成像应用由于E分子在聚集体状态下具有强发光特性,因此可应用于生物成像领域。
我们将所合成的E分子与生物样品共孵育,观察其发光情况。
实验结果表明,该E分子具有良好的生物相容性,可实现对生物样品的清晰成像。
(三)光治疗应用我们还研究了E分子在光治疗领域的应用。
《2024年近红外给体-受体AIE分子设计合成及光诊疗研究》范文
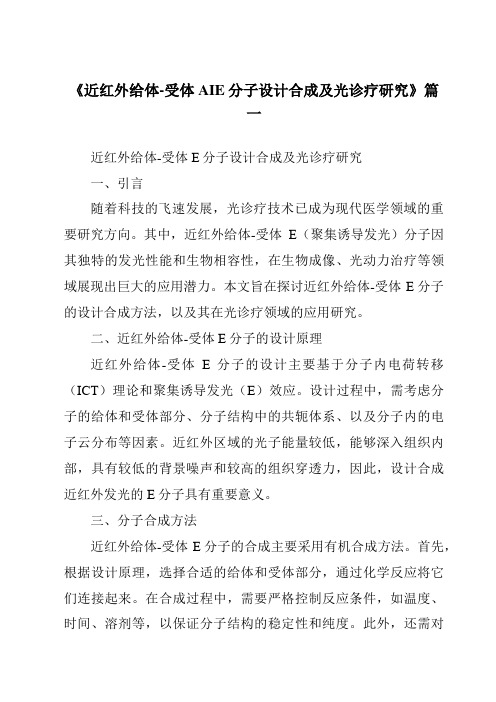
《近红外给体-受体AIE分子设计合成及光诊疗研究》篇一近红外给体-受体E分子设计合成及光诊疗研究一、引言随着科技的飞速发展,光诊疗技术已成为现代医学领域的重要研究方向。
其中,近红外给体-受体E(聚集诱导发光)分子因其独特的发光性能和生物相容性,在生物成像、光动力治疗等领域展现出巨大的应用潜力。
本文旨在探讨近红外给体-受体E分子的设计合成方法,以及其在光诊疗领域的应用研究。
二、近红外给体-受体E分子的设计原理近红外给体-受体E分子的设计主要基于分子内电荷转移(ICT)理论和聚集诱导发光(E)效应。
设计过程中,需考虑分子的给体和受体部分、分子结构中的共轭体系、以及分子内的电子云分布等因素。
近红外区域的光子能量较低,能够深入组织内部,具有较低的背景噪声和较高的组织穿透力,因此,设计合成近红外发光的E分子具有重要意义。
三、分子合成方法近红外给体-受体E分子的合成主要采用有机合成方法。
首先,根据设计原理,选择合适的给体和受体部分,通过化学反应将它们连接起来。
在合成过程中,需要严格控制反应条件,如温度、时间、溶剂等,以保证分子结构的稳定性和纯度。
此外,还需对合成得到的分子进行表征,如核磁共振、紫外-可见吸收光谱、荧光光谱等,以验证其结构和性能。
四、光诊疗应用研究1. 生物成像近红外给体-受体E分子在生物成像领域具有广泛的应用。
由于其发光强度高、背景噪声低、组织穿透力强等特点,使得其在细胞成像、组织成像等方面具有显著优势。
通过将E分子与生物分子或细胞标记物结合,可以实现高分辨率、高灵敏度的生物成像。
2. 光动力治疗光动力治疗是一种利用光敏剂和光照治疗肿瘤的方法。
近红外给体-受体E分子可作为光敏剂,在光照下产生单线态氧等活性氧物质,对肿瘤细胞产生杀伤作用。
此外,E分子的近红外发光性能有助于实时监测光动力治疗过程,为临床治疗提供有力支持。
五、实验结果与讨论通过合成不同结构的近红外给体-受体E分子,并对其在生物成像和光动力治疗中的应用进行研究。
Synthesis and characterization of novel systems fo

专利内容由知识产权出版社提供
专利名称:Synthesis and characterization of novel systems for guidance and vectorization of molecules of therapeutic interest towards target cells
发明人:Pascal Dumy,Marie-Christine Favrot,Didier Boturyn,Jean-Luc Coll
申请号:US10528320 申请日:20030919 公开号:US07531622B2 公开日:20090512
摘要:A method for preparing a grafted homodetic cyclopeptide forming a framework that defines a grafted upper face and grafted lower face, including synthesizing a linear peptide from modified or unmodified amino acids, some of which carry orthogonal protective groups; intramolecular cyclizing the resulting protetuting some or all of orthogonal protective groups with a protected precursor; and grafting at least one molecule of interest onto one and/or the other face of the framework via an oxime bond.
《近红外给体-受体AIE分子设计合成及光诊疗研究》范文

《近红外给体-受体AIE分子设计合成及光诊疗研究》篇一近红外给体-受体E分子设计合成及光诊疗研究一、引言近年来,随着科技的发展,分子材料在光诊疗领域的应用日益广泛。
其中,近红外给体-受体E(聚集诱导发光)分子因其独特的性质和潜在的应用价值,成为了研究的热点。
本文旨在探讨近红外给体-受体E分子的设计合成及其在光诊疗领域的应用研究。
二、近红外给体-受体E分子的设计思路近红外给体-受体E分子设计主要基于分子内电荷转移(ICT)和聚集诱导发光(E)原理。
设计思路主要包括选择合适的给体和受体,以及优化分子结构,以实现近红外区域的发光。
1. 给体和受体的选择给体和受体的选择对于分子的光学性质至关重要。
通常,给体选择具有强供电子能力的基团,如胺基、硫醇等;受体则选择具有强吸电子能力的基团,如氰基、硝基等。
此外,为了实现近红外区域的发光,需要选择能级适当的给体和受体,以实现有效的分子内电荷转移。
2. 分子结构的优化在确定给体和受体后,需要进一步优化分子结构,以实现E 效应。
这包括引入限制分子内旋转的基团、增加分子的共轭程度等。
此外,还需要考虑分子的溶解性和稳定性,以便于合成和实际应用。
三、近红外给体-受体E分子的合成方法近红外给体-受体E分子的合成主要采用有机合成方法。
具体步骤包括选择合适的原料、进行缩合反应、引入给体和受体等。
在合成过程中,需要注意反应条件、反应物的纯度等因素,以保证合成出高质量的E分子。
四、光诊疗应用研究近红外给体-受体E分子在光诊疗领域具有广泛的应用前景。
主要包括生物成像、光动力治疗和光热治疗等方面。
1. 生物成像近红外E分子具有较低的细胞毒性和较好的生物相容性,因此可用于生物成像。
通过将E分子与生物分子结合,可以实现对细胞、组织等的非侵入性成像。
此外,E分子的近红外发光特性使其能够减少背景噪声,提高成像质量。
2. 光动力治疗光动力治疗是一种利用光敏剂和光照共同作用来杀死癌细胞的治疗方法。
有机电致发光材料9-(1-萘基)-10-(2-萘基)蒽的合成及表征

有机电致发光材料9-(1-萘基)-10-(2-萘基)蒽的合成及表征杨杰;吕宏飞【摘要】采用9-溴蒽,1-萘硼酸,2-萘硼酸为原料,通过Suzuki偶联反应制备出9-(1-萘基)-10-(2-萘基)蒽(α,β-ADN).通过红外、核磁共振对其结构进行了表征.利用紫外和荧光光谱对其发光性能进行了研究.研究发现,α,β-ADN紫外可见吸收光谱有2个吸收带,分别归属为E带和B带,E带有2个吸收峰,波长分别为232 nm、262 nm;B带有3个吸收峰,其波长分别为359 nm、377 nm、398 nm,α,β-ADN的吸收带边为420 nm.采用401 nm的激发光激发,荧光发射峰值波长为440nm,属于蓝色荧光.【期刊名称】《黑龙江科学》【年(卷),期】2013(000)002【总页数】4页(P28-31)【关键词】α,β-AND;蓝光材料;Suzuki偶联;有机电致发光【作者】杨杰;吕宏飞【作者单位】黑龙江省科学院石油化学研究院,哈尔滨150040【正文语种】中文【中图分类】TQ241.5+5;TQ242.3有机电致发光器件(OLED)与其他显示器件相比具有自发光,不需要背景光源和滤光片,功耗低,低驱动电压(3~10 V),宽视角,响应速度快等特点,已成功应用于以手机为代表的小尺寸显示领域,并向电视、显示器等领域拓展[1-4]。
在全彩OLED平板显示领域,高效率和高纯度的红、蓝、绿三原色发光材料扮演着极其重要的角色。
其中蓝光材料不仅是蓝色光源的重要组成部分,而且还可作为绿光和红光材料能量转移掺杂的主体发光材料[5,6]。
迄今为止,人们一直致力于开发新型蓝色磷光或者荧光材料。
然而,蓝色荧光材料因为化合物本身的不稳定性以及色彩纯度问题,迟迟未能有所突破,与已经达到商业化要求的绿光材料相比,高效率和长寿命的蓝光材料与器件,特别是深蓝光材料与相应器件相对还比较缺乏[7,8],因此,新型深蓝光材料的研究与开发,成为目前OLED行业普遍关注的焦点。
新型不对称吡喃鎓方酸菁染料的晶体结构(英文)
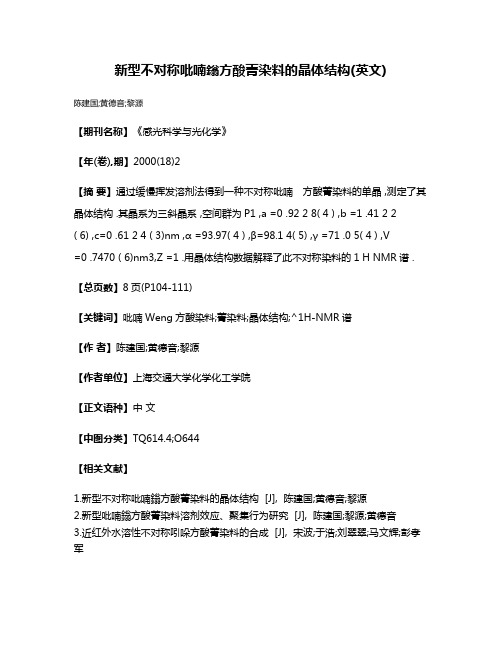
新型不对称吡喃鎓方酸菁染料的晶体结构(英文)
陈建国;黄德音;黎源
【期刊名称】《感光科学与光化学》
【年(卷),期】2000(18)2
【摘要】通过缓慢挥发溶剂法得到一种不对称吡喃方酸菁染料的单晶 ,测定了其晶体结构 .其晶系为三斜晶系 ,空间群为P1 ,a =0 .92 2 8( 4 ) ,b =1 .41 2 2 ( 6) ,c=0 .61 2 4 ( 3)nm ,α =93.97( 4 ) ,β=98.1 4( 5) ,γ =71 .0 5( 4 ) ,V
=0 .7470 ( 6)nm3,Z =1 .用晶体结构数据解释了此不对称染料的1 H NMR谱 .【总页数】8页(P104-111)
【关键词】吡喃Weng方酸染料;菁染料;晶体结构;^1H-NMR谱
【作者】陈建国;黄德音;黎源
【作者单位】上海交通大学化学化工学院
【正文语种】中文
【中图分类】TQ614.4;O644
【相关文献】
1.新型不对称吡喃鎓方酸菁染料的晶体结构 [J], 陈建国;黄德音;黎源
2.新型吡喃鎓方酸菁染料溶剂效应、聚集行为研究 [J], 陈建国;黎源;黄德音
3.近红外水溶性不对称吲哚方酸菁染料的合成 [J], 宋波;于浩;刘翠翠;马文辉;彭孝军
4.一种新型含肉桂酸酯结构的螺吡喃光致变色染料的合成与性能研究 [J], 申凯华; 崔东熏
因版权原因,仅展示原文概要,查看原文内容请购买。
一种光致变色水凝胶的合成与表征
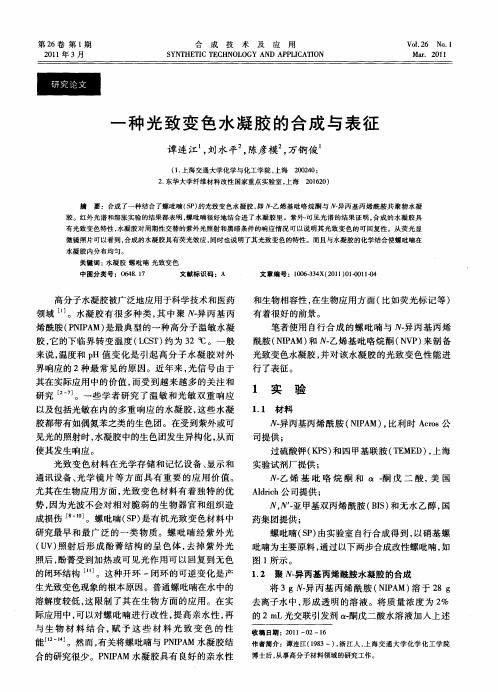
将 3gⅣ异丙 基丙烯酰胺 ( IA 溶 于 2 . NP M) 8g 去离子水 中, 形成透 明的溶液 。将 质量浓度为 2 %
的 2m L光 交联 引发 剂 O酮戊 二 酸水 溶 液加 入 上 述 t .
收稿 日期:2 1 - 2— 6 01 0 1 作者简介:谭连江 (9 3一) 浙 江人 , 海交通 大学化 学化工 学院 18 , 上
的闭环结 构 ¨ 。这种 开 环 一闭环 的 可逆 变 化 是产 生 光致变 色 现象 的根 本原 因 。普 通螺 吡 喃在水 中的 溶解 度较 低 , 限制 了其 在 生 物 方 面 的应 用 。在 实 这
实验试 剂 厂提供 ; Ⅳ. 烯 基 吡 咯 烷 酮 和 t 酮 戊 二 酸 , 国 乙 l t一 美 Ad c 司提供 ; lrh公 i
以及 包括 光敏 在 内 的多 重 响 应 的水 凝 胶 , 些 水 凝 这
1 实 验
1 1 材料 .
胶都带有如偶氮苯之类的生色团。在受到紫外或可
见光 的照 射 时 , 水凝 胶 中 的生 色 团发生 异构 化 , 而 从
Ⅳ一 丙基 丙烯 酰胺 ( IA , 异 NP M) 比利 时 A rs co 公
第2 6卷 第 1期 2 1 年 3月 01
合
成
技
术
及
应
用
Vo - 6 No 1 l2 .
M a . 201 r 1
S YNT C HEnC TE HN0L OGY AN AP U C D P A nON
一
种 光 致变 色水 凝 胶 的合 成 与表 征
谭连 江 刘水 平 陈彦模 万锕俊 , , ,
Ⅳ, Ⅳ一 亚甲基双丙烯酰胺 ( I) BS 和无水 乙醇 , 国
高温固相法制备ZnMoO_(4):Eu^(3+)荧光粉及其发光性质研究

(b)
5D0 → 7F2
ZnMoO4:xEu3+ λem=617nm
x=0.01 x=0.02 x=0.03 x=0.04 x=0.05
ZnMoO4 在此条件下成功地被制备出来,并且没有杂相生成。 此外,Eu3+ 的引入并没有引起基质晶格明显的改变,这是因 为 Zn2+(0.88Å)和 Eu3+(1.087Å)离子半径和配位环境相似, 以及掺杂浓度并不是很高,所以稀土 Eu3+ 能够成功掺杂到基 质 ZnMoO4 中。
收稿日期 :2021–03–16 基金项目 :2021 年 度 吉 林 省 教 育 厅“ 十 四 五 ” 科 研 规 划 项 目
(JJKH20210542KJ) 作者简介 :吴宏越(1987—),男,吉林龙井人,讲师,主要研究方
向为稀土发光材料。 通讯作者 :李琳琳(1987—),女,吉林通化人,副教授,主要研究
此仪器装备的氙灯是 150W。所有测试都在室温下进行。 ·95·
第47卷第6期
2021年6月
研究与开发
Research and Development
化工设计通讯
Chemical Engineering Design Communications
3 结果与讨论 3.1 所制备样品的物相分析
图 1 为 最 大 掺 杂 浓 度 样 品 ZnMoO4 :0.05Eu3+ 和 ZnMoO4 标准粉末 X 射线衍射数据(PDF#72-1486)的 XRD 图谱。将 合 成 的 样 品 ZnMoO4 :0.05Eu3+XRD 衍 射 峰 图 谱 与 ZnMoO4 的标准衍射图谱进行对比,可以看出两者匹配得很好,得知
在近些年的研究中,制备荧光粉的方法日渐多样化,目 前常用的方法有 :高温固相合成法、微波热合成法、共沉淀 合成法等。不同的制备条件和方法会直接影响制备出发光材 料的使用效果和决定它是否可以普遍应用。本文选用的是高 温固相法来制备荧光粉,应用该方法制备的荧光粉优点很多 性能稳定,发光强度高,且制备方法既简单环保又利于工业 上大批生产,因此得到了广泛应用 [4]。
不同形貌羟基磷灰石纳米颗粒的可控合成及其对骨肉瘤细胞生长的影响
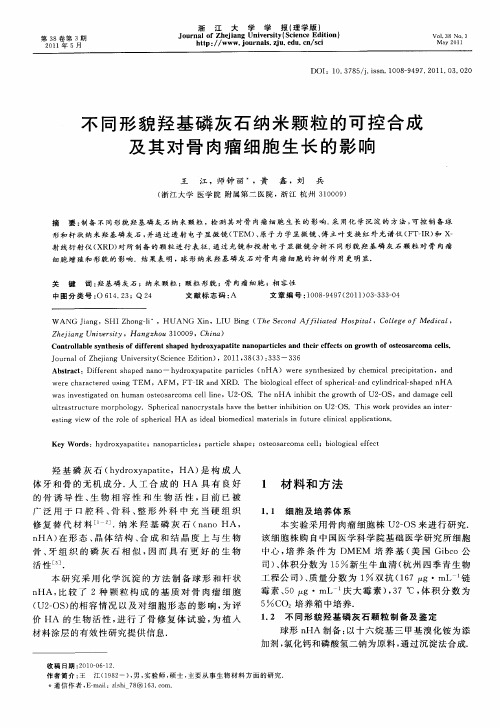
关 键 词 :羟 基 磷 灰 石 ;纳 米颗 粒 ;颗粒 形貌 ;骨 肉瘤 细 胞 ;相 容 性
中图 分 类 号 :O 614.23;Q 24
文献 标 志 码 :A
文 章 编 号 :1008—9497(2011)03—333—04
W ANG Jiang,SHI Zhong—li ,HUANG Xin,LIU Bing (The Second Affiliated Hospital,College of M edical, ZheJiang University,H angzhou 310009,China)
K ey W ords:hydroxyapatite;nanoparticles;particle shape;osteosarcom a cell; biological effect
羟 基 磷 灰 石 (hydroxyapatite,HA)是 构 成 人 体 牙 和 骨 的无 机成 分 .人 工 合 成 的 HA 具 有 良好 的 骨 诱 导 性 、生 物 相 容 性 和 生 物 活 性 ,目前 已 被 广 泛 用 于 口 腔 科 、骨 科 、整 形 外 科 中 充 当 硬 组 织 修 复 替 代 材 料 l】 ].纳 米 羟 基 磷 灰 石 (nano HA, nHA)在 形 态 、晶 体 结 构 、合 成 和 结 晶 度 上 与 生 物 骨 、牙 组 织 的 磷 灰 石 相 似 ,因 而 具 有 更 好 的 生 物 活 性 E3].
不 同形 貌 羟 基 磷 灰 石 纳 米 颗 粒 的 可 控 合 成 及 其 对 骨 肉瘤 细 胞 生 长 的 影响
王 江 ,师钟 丽 ,黄 鑫 ,刘 兵
(浙 江 大 学 医 学 院 附 属 第 二 医 院 ,浙 江 杭 州 310009)
不对称合成化学
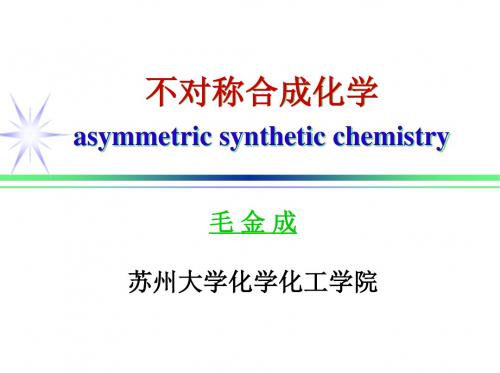
Different grafting ways
Interaction Absorption
The confinement effect of the pore and surface
Various factors
Others
离子液体 反应控制相转移 温控相转移 等等
The examples in detail
P.-N. Liu, P.-M. Gu, J.-G. Deng, Y.-Q. Tu, Y.-P. Ma, Eur. J. Org. Chem. 2005, 15, 3221-3227
A Recyclable Fluorous (S)Pyrrolidine Sulfonamide Promoted Direct, Highly Enantioselective Michael Addition of Ketones and Aldehydes to Nitroolefins in Water
Asymmetric Iridium-Catalyzed Allylic Alkylation
A. Alexakis, D. Polet, Org. Lett. 2004, 6, 3529-3532
Synthesis and Characterization of Li(Li0.23Mn0.47Fe0.2Ni0.1)O2 Cathode Material for Li-ion Batteries

retention
Li(Li0.23Mn0.47Fe0.2Ni0.1)O2 compound synthesized easily at low temperature by the proposed citric acid assisted sol-gel process is a promising cathode material for Li-ion batteries.
57
EP
TE D
(1−z)Li[Li1/3Mn2/3]O2−(z)Li[Mn0.5−yNi0.5−yCo2y]O2
M AN U
[11]. to
2
Li2MnO3–LiCo1/3Ni1/3Mn1/3O2
SC
The 4.0~4.5V)
storage power station applications. Therefore, new cathode materials with high capacity, low cost and environment friendly have been investigated thoroughly in recent years [1]. Among them the layered Li2MnO3-based solid solution materials have attracted more attention of the researchers, such as Li2MnO3-LiMO2 (M = Ni, Cr and Co) [2–5], LiNiO2–Li2MnO3–LiCoO2 [8], and [6], Li2MnO3–LiNi1−xCoxO2 [7], [9], [10], above-mentioned
不同溶剂中的荧光

Journal of Luminescence 124(2007)365–369Synthesis and fluorescence study of sodium-2-(40-dimethyl-aminocinnamicacyl)-3,3-(10,30-alkylenedithio)acrylateZhenjun Si,Yun Shao Ã,Chunxia Li,Qun LiuSchool of Chemistry,Northeast Normal University,Changchun 130024,PR China Received 9January 2006;received in revised form 5April 2006;accepted 14April 2006Available online 5June 2006AbstractWe synthesized two new compounds:Sodium 2-(40-dimethyl-aminocinnamicacyl)-3,3-(10,30-ethyl-enedithio)acrylate (STAA-1)and Sodium 2-(40-dimethyl-aminocinnamicacyl)-3,3-(10,30-propylenedithio)acrylate (STAA-2).The maximum absorption of these compounds ranges from 460to 520nm with different molecular structures in different solvents.Meanwhile,the emission peak of these compounds arranges from yellow (510nm)to red (605nm).The emission spectra show red shift according to the strength of the hydrogen bonding property of the solvent.But the absorption spectra do not show clearly relationship with the strength of the hydrogen bonding property of the solvent.The Stoke shift of the compounds ranges from 42to 102nm.It changes in the following order,EtOH 4H 2O 4DMF,and STAA-14STAA-2in the same solvent.The fluorescent quantum yield of STAA-1was measured to be 7.12%with quinine sulphate as the standard compound in ethanol.Furthermore,the relationship of the fluorescence of STAA-1with pH (ranges form 4to 14)in water (c ¼$10À4)was studied to make sure that these compounds could be used as proton sensors.r 2006Elsevier B.V.All rights reserved.PACS:07.07.Df;78.55.Àm;81.20.ÀnKeywords:Proton sensor;Fluorescence quantum yield;Synthesis1.Introductionp -Conjugated organic compounds have emerged in the past two decades as a promising class of materials for potential applications in photonics and optoelectronics.Lots of active materials used in these fields are the derivatives of aniline.These materials are used in non-linear optics [1],photovoltaic cell [2],light emitting devices [3],and optically pumped lasers [4].The photo-physical properties of the aromatic amino acids depend on the status of the amino and carboxyl groups although they are separated form chromophore by some methylene groups [5,6]or other groups [7,8],the quenching efficiency is controlled by the orientation of the quenching groups and the process is distance dependent.The rotamer model of fluorescence decays of aromatic amino acids has beensupported by some investigations [9–11]that included studies of constrained tryptophan [12,13],tryptophan in protein crystals [14]and tryptophan in peptides with defined secondary structure [15].For several decades,fluorescence spectroscopy has been widely used for the detection and analysis of different analytes [16,17].Wavelength-ratiometric [18,19],fluores-cence lifetime-based sensing [20,21]and polarization assays [22]are some techniques available for the detection and analysis of analytes by fluorescence spectroscopy.Fluores-cence techniques for pH sensor have been used most of the time with enzymes and proteins [23].Despite some promising results,enzymes and proteins show some stability problems against organic solvents and heat.In contrast,synthetic organic probes show high stability and flexibility due to the versatility of the organic synthesis.Modification of the affinity for the analyte,of the wavelength of emission of the probes and of the immobilization of the probes is in support for the building of a sensor./locate/jlumin0022-2313/$-see front matter r 2006Elsevier B.V.All rights reserved.doi:10.1016/j.jlumin.2006.04.005ÃCorresponding author.Tel.:+8604315099667.E-mail address:*****************.cn (Y.Shao).In this essay,we introduce two compounds we synthe-sized,present photo-physical properties of STAA-1and STAA-2in different solvents in order to study the solvent polarity effects on the photo-physical property,further-more we present the photo-physical property of STAA-1in water at different pH value.Up to now,the latest report is about thefluorescent molecular,which contains dithioace-tals as we know.2.Experiment2.1.MaterialsEthanol(EtOH),N,N-dimethylformamide(DMF),car-bon bisulphide(CS2),acetic acid ethyl ester,potassium carbonate(K2CO3),1,2-dibromoethane,1,3-dibromopro-pane were used as supplied,but only after checking the purityfluorometrically in the wavelength region of interest. The solvent,water,was distilled twice in vacuum and tested for the absence of any emission in the wavelength regions studied.Absorption and emission spectra were recorded on Shimadzu Model3100spectrometer and Hitachi Spectro-photometer model F-4500,respectively.Spectrum correc-tion has been performed in order to measure a true spectrum by eliminating instrumental response such as wavelength characteristics of the monochromators or detectors.For all measurements,the sample concentration was restricted at$10À5mol/L in order to avoid the aggregation problems such as self-quenching.The cut-off filter was used to prevent scattering of excitation beam.The relativefluorescent quantum yield of STAA-1was mea-sured with quinine sulphate as the standard compound in sulphuric acid according to the following equation[24]:f fx¼n2x ÁF xÁf fstd=n2stdÁF std.(1)2.2.Preparation of materials(Scheme1)2.2.1.3-(10,30)-Dithiolan-2-ylidene-pentane-2,4-dione IaAnhydrous K2CO3(13.8g)and6.25mL acetic acid ethylester were added to100mL DMF in250mLflask,themixture was stirred0.5h and cooled to0–51C,3.5mL CS2was added,the stirring was continued for another0.5h,then 4.75mL1,2-Dibromo-ethane was added to themixture.The mixture was poured into a400mLflask with200g ice after it was stirred overnight.The deposition wasfiltrated,the yield of Ia was98%.(The data ofcharacterization is in Refs.[25,26].)2.2.2.Sodium-2-(40-dimethyl aminocinnamicacyl)-3,3-(10,30-propylenedithio)acrylate STAA-12.2mmol4-Dimethylamino-benzaldehyde and2.0mmolcompound Ia were added into10mL EtOH in a25mLflask,then2.0mmol EtONa was dropped into theflask,then stirred at701C,detected with TLC until thecompletion of the reaction.Then the yellow solid wasfiltrated and collected after the mixture was cooled to roomtemperature.The yield was70%.The synthesis of Ib and STAA-2is similar to that of Ia andSTAA-1,respectively.2.3.Characterization of compounds STAA-1and STAA-2STAA-1:Yellow solid,yield:85%,m.p.210–2111C1HNMR:7.370(2H,d,J¼21ArH),7.234(2H,d,J¼3.8,H),6.670(2H,d,J¼3.8,H),6.549(1H,d,J¼21,ArH),3.36(4H,tri,J¼6,–SCH2–),2.945(6H,s,–NC2H6);IR n(cmÀ1)1579,1524,1348,1182,3425.STAA-2:Green-yellow solid,yield:83%,m.p.207–208.51C1HNMR7.407(2H,d,J¼22ArH),7.301(1H,d,J¼4,H), 6.797(1H,d,J¼4,H),6.718(2H,d,J¼22,ArH), 2.965(6H,s,–NC2H6),O OOC2H5SS( ) nO OONaCS2BrBr++DMF23( ) nNO OONaSS( ) nO OOC2H5SS( ) n+ONn = 1,2II a(a)(b)I II II bIScheme1.(a)Synthesis of compounds STAA-1and STAA-2and(b)the configurations of compounds STAA-1and STAA-2.Z.Si et al./Journal of Luminescence124(2007)365–3693662.787(4H,m,J¼17,–SCH2–), 2.019(2H,m,J¼17,–CH2–);IR n(cmÀ1)1574,1523,1365,3442,1183. 3.Results and discussion3.1.Absorption spectraThese compounds show two strong main peaks between 256and446nm(Fig.1).The higher energy but weaker absorption band lies at256nm does not show obvious shift according to the change of solvents or the ring size of dithioacetals.It is assigned to be the absorption of the derivatives of benzene.The structureless broad absorption spectra whose peak lies in the range of309–446nm(Fig.1) can be affected by both the size of the ring of dithioacetals and the property of the paring the position with benzene derivatives,we can assign the lower energy bands as1La[26].It shows red-shift according to the strength of the hydrogen bonding property of the solvent (Table1).The two peaks show the largest span when those compounds are in water,and smallest in DMF unlike other benzene derivatives[7].And the lower energy band of the compound STAA-2shows somewhat red-shift compared with that of the compound STAA-1in Water or EtOH,but it only shows a little difference in the extent of the half-wave without any other difference.It is evidenced that the absorption property of these compounds is associated with the property of solvent and the ring size of the dithioacetals in the molecular.As for the property that these two compounds are strongly soluble in polar solvents and weakly soluble in non-polar solvents,we can assume them being polar in ground state.So from the aprotic solvent effect,the lower energy band can be attributed to intramolecular charge transfer electronic states.3.2.Emission spectra at room temperatureBoth of the compounds exhibit only one anomalous emission band in different polar solvents.The structureless emission band of the two compounds shows a large red-shift from non-polar to polar solvents at about510–605nm wavelength region(Figs.2b and3).The maximum emission point of compound STAA-2is located at a lower energy region than that of compound STAA-1.So the fluorescence of these type of compounds is affected by not only the property of solvents but also the size of the ring of dithioacetals.As a result,the lowest energy emission is atFig.1.The absorption spectra of compounds(a)STAA-1and(b)STAA-2in different solvents;rectangle—DMF,circle—EtOH,triangle—water.Fig.2.Thefluorescent spectra of compounds(a)STAA-1and(b)STAA-2in different solvents;rectangle—DMF,circle—EtOH,triangle—water.Z.Si et al./Journal of Luminescence124(2007)365–369367605nm for compound STAA-2when in water,and the highest energy emission is at 510nm for compound STAA-1when in DMF (Table 1).Because the emission of compound STAA-1in EtOH is the strongest for these compounds,the fluorescent quantum efficiency of STAA-1was measured to be 7.12%with quinine sulphate as the standard compound in ethanol at room temperature.It is interesting to note that the Stoke shift of these compoundsranges form 42to 102nm in different solvents.And the Stoke shift of these compounds changes in the following order:EtOH 4Water 4DMF for both of the compounds,and STAA-14STAA-2in the same solvent.Although we cannot offer a more thorough explanation for this phenomenon,we can assign that this might be the result of the special molecular structure.3.3.Emission spectra of STAA-1in water at room temperatureIn order to study the relationship between the fluores-cence of the compounds STAAs and the pH value of water,we have detected the emission spectrum of STAA-1(it’s fluorescence is stronger than the other one’s)in water with different pH value.We found that the emission spectrum of STAA-1was affected by the pH value dramatically (Fig.4).The emission intensity increases according to the increasing of pH value when the pH value is between 7.5and 9.0.The maximum intensity appears when the pH value is 9.0.The intensity of fluorescence lowers slightly with further increase of the value of pH.We attribute the decrease to the effect of increased concentration of salt (Fig.4b ).If the value of pH is lower than 7.5,the fluorescence of STAA-1disappears (Fig.4a ).So it is possible that the compounds STAAs could be used as the sensors of proton in the future.Though we do not know the exact principle of the quenching of the fluorescence ofTable 1Absorption and fluorescence data of Sodium-2-(40-dimethylaminocinnamicacyl)-3,3-(10,30-alkylenedithio)acrylate (STAA-1,STAA-2)at room temperature CompoundsAbsorption (l max )Fluorescence DMFEtOHH 2ODMF EtOH H 2O l max (em)l max (ex)l max (em)l max (ex)l max (em)l max (ex)STAA-1407428433510441550448591497STAA-2396429446514463559460605520Fig.4.(a)The fluorescent spectrum of compound DTAA-1in water with different pH (c ¼$10À4mol/L)and (b)plot of maximum intensity fluorescence of compound DTAA-1in water vs.pH.0.00.20.40.60.81.0A b s o r p t i o n (a .u .)Wavelength (nm)Fig.3.The fluorescent spectrum of compound DTAA-1in the mixed solvent of DMF and EtOH with different ratio;filled rectangle—EtOH,opened rectangle—20%DMF+80%EtOH,filled circle—40%DMF+60%EtOH,opened circle—60%DMF+40%EtOH,filled triangle—80%DMF+20%EtOH,opened triangle—DMF.Z.Si et al./Journal of Luminescence 124(2007)365–369368this type of compounds,we attributed this phenomenon to the proton trapping of the dimethylaniline group in the molecules.When the pH is lower than7.5,the donor groups(ÀN(CH3)2)in the molecules trap the protons in the solvent and become another group,ammonium (À+NH(CH3)2),then the donors in the molecules dis-appear.When the electron cannot be transported to the acceptor group in the molecular,thefluorescence of STAA-1could not be detected any more.4.ConclusionIn summary,we synthesized two compounds by a two-step reaction in high yields(480%).The unique molecular structure awards these compounds special photo-physical property.The emission peak of these compounds shifts according to the hydrogen bonding property of the solvent. The Stoke shift changes as EtOH4Water4DMF for both of the compounds.But there is no obvious relationship between absorption spectrum and the property of the solvent.Thefluorescent quantum efficiency of STAA-1was measured to be7.12%at room temperature.Furthermore, by studying the relationship between thefluorescent property of STAA-1and the pH value of water,we found the compound STAA-1could be the proton sensor in further studies.References[1]B.A.Reinhardt,et al.,Chem.Mater.10(1998)1863.[2]T.Horiuchi,H.Miura,K.Sumioka,S.Uchida,J.Am.Chem.Soc.126(2004)12218.[3]C.Chen,Chem.Mater.16(2004)4389.[4]Y.Huang,T.Cheng,F.Li,C.Huangect,J.Phys.Chem.B106(2002)10041.[5]J.M.Beechem,L.Brand,Annu.Rev.Biochem.54(1981)43.[6]J.B.A.Ross,ws,K.W.Roussland,H.R.Wyssbrob,Topicsoffluorescence spectroscopy,in:kowicz(Ed.),Biochemical Applications,vol.3,Plenum Press,New York,1992.[7]P.R.Bangal,S.Chakravorti,J.Photochem.Photobiol.A:Chem.116(1998)191.[8]D.F.Hoelzl Wallach,T.L.Steck,Anal.Chem.35(1963)1035.[9]B.S.Hudson,J.M.Hutson,J.Phys.Chem.103(1999)2227.[10]M.C.Chang,J.W.Petrich,D.B.McDonald,G.R.Fleming,J.Am.Chem.Soc.105(1983)3819.[11]R.J.Robbins,G.R.Fleming,G.S.Beddard,G.W.Robinson,P.J.Thistlethwait,G.J.Wolfe,J.Am.Chem.Soc.102(1980)6271.[12]L.Tilstra,M.C.Sattler,W.Cherry,M.D.Barkley,J.Am.Chem.Soc.112(1990)9176.[13]M.R.Eftink,Y.Jia,D.Hu,J.Phys.Chem.99(1995)5713.[14]T.E.S.Dahms,J.K.Willis,A.G.Szabo,J.Am.Chem.Soc.117(1995)3221.[15]A.H.A.Clayton,W.H.Sawyer,Biophys.J.76(1999)3235.[16]D.Cui,X.Qian,F.Liu,R.Zhang,Org.Lett.6(2004)2757.[17]T.Morii,K.I.Sugimoto,K.Makino,Ma.Otsuka,K.Imoto,Y.Mori,J.Am.Chem.Soc.124(2002)1138.[18]K.Aslan,kowicz,C.D.Geddes,Anal.Chem.77(2005)2007.[19]D.Roll,J.Malicka,I.Gryczynski,Z.Gryczynski,kowicz,Anal.Chem.75(2003)3440.[20]E.Kuwana,E.M.Sevick-Muraca,Anal.Chem.75(2003)4325.[21]C.M.Maragos,M.E.Jolley,R.D.Plattner,M.S.Nasir,J.Agric.Food Chem.49(2001)596.[22]C.M.Maragos,R.D.Plattner,J.Agric.Food Chem.50(2002)1827.[23]H.Frenkel-Mullerad, D.Avnir,J.Am.Chem.Soc.127(2005)8077.[24]Y.Cui,et al.,Chinese Journal of Analytical Chemistry4(1986)616.[25]E.B.Choi,I.K.Youn,C.S.Pak,Synthesis1984(1984)797.[26]Q.Liu,Z.Zhu,et al.,J.Liaoning Normal Univ.18(1995)304(in Chinese).Z.Si et al./Journal of Luminescence124(2007)365–369369。
量子点荧光纳米材料的化学合成及其在潜手印显现中的应用

术 已引起 了研究 者 的浓 厚兴趣 。合成 出高 质量 、 高荧光 性能 的量子 点是 拓展 其 在手 印显 现 方 面广 泛应 用 的 前提条 件 。本文 针对量 子点荧 光纳 米材料 的化学 法合 成及其 在潜手 印显 现领域 中的应 用进展 进行 了较 为详
尽 的归纳 和总结 。
关键 词 :量子点 , 手 印显 现 , 潜 在手 印 , 纳米 材料
中 图分类号 : D 9 1 8
C h e mi c a l S y n t h e s i s o f Qu a n t u m D o t s F l u o r e s c e n t Na n o ma t e r i a l s
,
YI Ku i . y u ,FENG Ya n g ,LI Ta o ,ZHANG Ni n g
( 1 D e p a r t m e n t o f T r a c e E x a m i n a t i o n T e c h n o l o g y C h i n a C i r m i n a l P o l i c e C o l l e g e , S h e n y a n g 1 1 0 0 3 5, L i a o n i n g , C h i n a ;
a n d Th e i r Ap p l i c a t i o n s i n De v e l o p me nt o f La t e n t Fi n g e r p r i n t s
GAO Ya n g — c h e n ,W ANG Me ng ,ZHANG Xi a o — me i ‘ YANG Mi n g— f e i ,
甘露糖衍生物的合成及磁性荧光纳米复合粒子的表面修饰
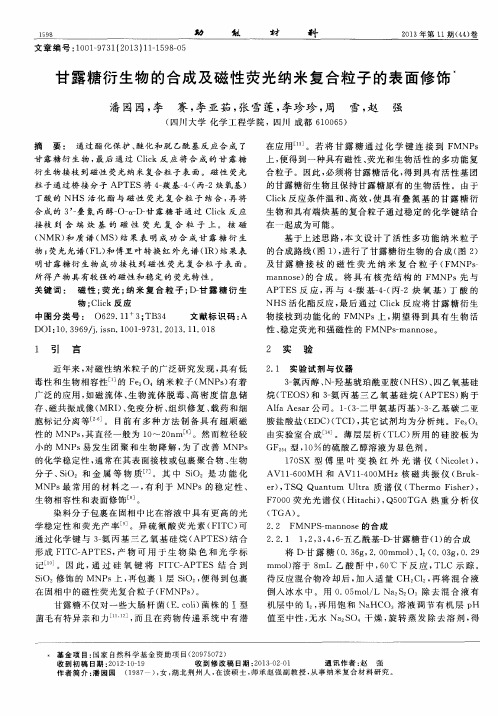
1 7 0 S X型傅里 叶变换红 外光谱 仪 ( Ni c o l e t ) ,
AV1 1 - 6 0 0 MH 和 AV1 1 - 4 0 0 MHz核 磁 共 振 仪 ( B r u k —
e r ) , TS Q Qu a n t u m Ul t r a质 谱 仪 ( T h e r mo F i s h e r ) , F 7 0 0 0荧 光 光 谱 仪 ( Hi t a c h i ) , Q5 0 0 T GA 热 重 分 析仪
的化学 稳定性 , 通 常在其 表面接 枝或包 裹 聚合 物 、 生 物 分子、 S i O 和 金 属 等 物 质[ 7 ] 。其 中 S i O 是 功 能 化
MNP s 最 常用 的材 料 之 一 , 有 利 于 MNP s的 稳 定 性 、 生物相 容性 和表 面修饰 _ 8 j 。 染 料分子 包裹 在 固相 中比在 溶液 中具 有更 高 的光 学 稳定 性 和荧 光 产 率 l _ 9 ] 。异 硫 氰 酸 荧 光 素 ( F I T C) 可
近年来 , 对 磁性纳 米粒 子 的广 泛研究 发 现 , 具 有 低 毒性 和生 物相 容性1 ] 的F e 。 O 纳 米粒 子 ( MN P s ) 有 着
2 实 验
2 . 1 实 验 试 剂 与 仪 器
广 泛 的应 用 , 如磁 流体 、 生 物流 体 脱毒 、 高 密度 信 息 储 存、 磁共 振成像 ( MRI ) 、 免疫 分析 、 组织 修 复 、 载 药 和细 胞标 记 分离 等 ] 。 目前 有 多种 方 法 制 备具 有 超 顺 磁
物; 荧光 光谱 ( F L ) 和傅 里叶 转换 红 外光 谱 ( I R) 结果 表
明甘 露 糖 衍 生 物 成 功 接 枝 到 磁 性 荧 光 复 合 粒 子 表 面 。 所 得 产 物 具 有 较 强 的磁 性 和 稳 定 的 荧光 特 性 。 关键 词 : 磁 性; 荧光 ; 纳米复合粒 子; D - 甘 露 糖 衍 生
生物质焦油制备的碳量子点
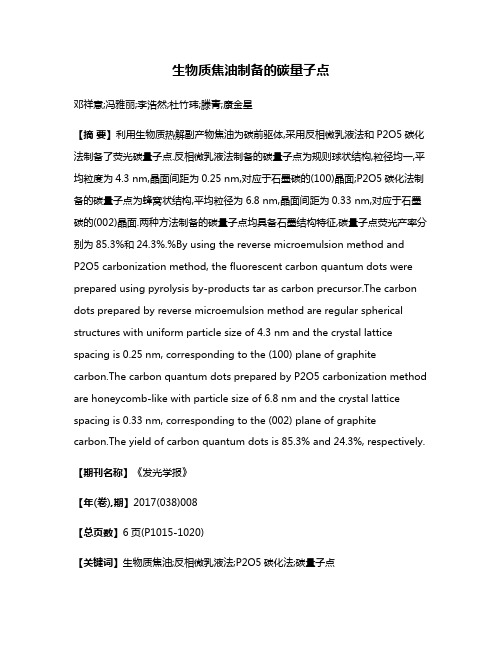
生物质焦油制备的碳量子点邓祥意;冯雅丽;李浩然;杜竹玮;滕青;康金星【摘要】利用生物质热解副产物焦油为碳前驱体,采用反相微乳液法和P2O5碳化法制备了荧光碳量子点.反相微乳液法制备的碳量子点为规则球状结构,粒径均一,平均粒度为4.3 nm,晶面间距为0.25 nm,对应于石墨碳的(100)晶面;P2O5碳化法制备的碳量子点为蜂窝状结构,平均粒径为6.8 nm,晶面间距为0.33 nm,对应于石墨碳的(002)晶面.两种方法制备的碳量子点均具备石墨结构特征,碳量子点荧光产率分别为85.3%和24.3%.%By using the reverse microemulsion method andP2O5 carbonization method, the fluorescent carbon quantum dots were prepared using pyrolysis by-products tar as carbon precursor.The carbon dots prepared by reverse microemulsion method are regular spherical structures with uniform particle size of 4.3 nm and the crystal lattice spacing is 0.25 nm, corresponding to the (100) plane of graphite carbon.The carbon quantum dots prepared by P2O5 carbonization method are honeycomb-like with particle size of 6.8 nm and the crystal lattice spacing is 0.33 nm, corresponding to the (002) plane of graphite carbon.The yield of carbon quantum dots is 85.3% and 24.3%, respectively.【期刊名称】《发光学报》【年(卷),期】2017(038)008【总页数】6页(P1015-1020)【关键词】生物质焦油;反相微乳液法;P2O5碳化法;碳量子点【作者】邓祥意;冯雅丽;李浩然;杜竹玮;滕青;康金星【作者单位】北京科技大学土木与资源工程学院,北京 100083;北京科技大学土木与资源工程学院,北京 100083;中国科学院过程工程研究所生化工程国家重点实验室,北京 100190;中国科学院过程工程研究所生化工程国家重点实验室,北京100190;北京科技大学土木与资源工程学院,北京 100083;北京科技大学土木与资源工程学院,北京 100083【正文语种】中文【中图分类】O613.71碳量子点是以碳为骨架结构、尺寸小于10 nm的荧光纳米颗粒[1],化学稳定性高,无光漂白和光眨眼现象。
铝离子与羟基功能化荧光碳点的相互作用
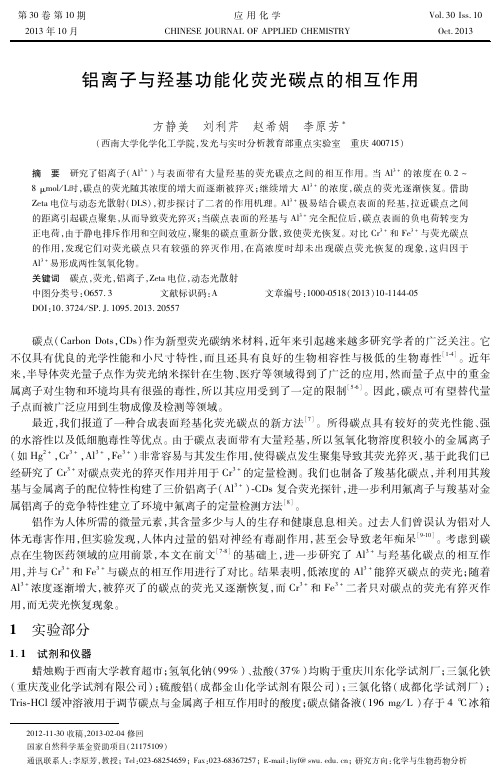
第30卷第10期应用化学Vol.30Iss.102013年10月 CHINESEJOURNALOFAPPLIEDCHEMISTRY Oct.2013铝离子与羟基功能化荧光碳点的相互作用方静美 刘利芹 赵希娟 李原芳(西南大学化学化工学院,发光与实时分析教育部重点实验室 重庆400715)摘 要 研究了铝离子(Al3+)与表面带有大量羟基的荧光碳点之间的相互作用。
当Al3+的浓度在0 2~8μmol/L时,碳点的荧光随其浓度的增大而逐渐被猝灭;继续增大Al3+的浓度,碳点的荧光逐渐恢复。
借助Zeta电位与动态光散射(DLS),初步探讨了二者的作用机理。
Al3+极易结合碳点表面的羟基,拉近碳点之间的距离引起碳点聚集,从而导致荧光猝灭;当碳点表面的羟基与Al3+完全配位后,碳点表面的负电荷转变为正电荷,由于静电排斥作用和空间效应,聚集的碳点重新分散,致使荧光恢复。
对比Cr3+和Fe3+与荧光碳点的作用,发现它们对荧光碳点只有较强的猝灭作用,在高浓度时却未出现碳点荧光恢复的现象,这归因于Al3+易形成两性氢氧化物。
关键词 碳点,荧光,铝离子,Zeta电位,动态光散射中图分类号:O657.3 文献标识码:A 文章编号:1000 0518(2013)10 1144 05DOI:10.3724/SP.J.1095.2013.20557碳点(CarbonDots,CDs)作为新型荧光碳纳米材料,近年来引起越来越多研究学者的广泛关注。
它不仅具有优良的光学性能和小尺寸特性,而且还具有良好的生物相容性与极低的生物毒性[1 4]。
近年来,半导体荧光量子点作为荧光纳米探针在生物、医疗等领域得到了广泛的应用,然而量子点中的重金属离子对生物和环境均具有很强的毒性,所以其应用受到了一定的限制[5 6]。
因此,碳点可有望替代量子点而被广泛应用到生物成像及检测等领域。
最近,我们报道了一种合成表面羟基化荧光碳点的新方法[7]。
所得碳点具有较好的荧光性能、强的水溶性以及低细胞毒性等优点。
- 1、下载文档前请自行甄别文档内容的完整性,平台不提供额外的编辑、内容补充、找答案等附加服务。
- 2、"仅部分预览"的文档,不可在线预览部分如存在完整性等问题,可反馈申请退款(可完整预览的文档不适用该条件!)。
- 3、如文档侵犯您的权益,请联系客服反馈,我们会尽快为您处理(人工客服工作时间:9:00-18:30)。
13Journal of Inorganic andOrganometallic Polymers and MaterialsISSN 1574-1443Volume 23Number 3J Inorg Organomet Polym (2013)23:771-778DOI 10.1007/s10904-013-9825-xSynthesis, Characterizations and Luminescent Properties of TwoCadmium(II) Coordination Polymers Derived from Bis(Benzimidazole)-Based LigandsShu Lin Xiao, Li Qin, Cui Hong He, Xu Du & Guang Hua CuiYour article is protected by copyright and all rights are held exclusively by Springer Science +Business Media New York. This e-offprint is for personal use only and shall not be self-archived in electronic repositories. If you wish to self-archive your article, please use the accepted manuscript version for posting on your own website. You may further depositthe accepted manuscript version in any repository, provided it is only made publicly available 12 months after official publicationor later and provided acknowledgement is given to the original source of publicationand a link is inserted to the published article on Springer's website. The link must be accompanied by the following text: "The final publication is available at ”.13COMMUNICATIONSynthesis,Characterizations and Luminescent Properties of Two Cadmium(II)Coordination Polymers Derived from Bis(Benzimidazole)-Based LigandsShu Lin Xiao •Li Qin •Cui Hong He •Xu Du •Guang Hua CuiReceived:5September 2012/Accepted:11January 2013/Published online:22January 2013ÓSpringer Science+Business Media New York 2013Abstract Two Cd(II)coordination polymers,namely,{[Cd(L1)(chdc)]Á(CH 3CN)Á(H 2O)2}n (1)and [Cd(L 2)Cl 2]n (2)(L1=1,4-bis(5,6-dimethylbenzimidazole-1-ylmethyl)benzene,L2=1,2-bis(5,6-dimethylbenzimidazole-1-ylme thyl)benzene,H 2chdc =1,4-cyclohexanedicarboxylic acid )have been obtained by hydrothermal methods and char-acterized by IR,TG,elemental analysis,XPRD and single crystal structure analysis.The Cd(II)atom has a distorted octahedral coordination geometry in 1and a roughly square-pyramidal coordination geometry in 2.Polymer 1possesses a two-dimensional (2D)flat (6,3)network con-structed with L1and chdc bridging ligands.Polymer 2displays a one-dimensional (1D)loop-like chain structure bridged by chlorine anions and L2ligand,which is further arranged into a 2D supramolecular layer through face-to-face p –p stacking interactions.The luminescent properties of the polymers 1and 2were investigated in the solid-state.Keywords Bis(benzimidazole)ÁCadmium(II)coordination polymer ÁCrystal structure ÁLuminescent properties 1IntroductionThe last two decades have shown impressive progress in the design and synthesis of a new type of material generallyreferred to as metal-organic frameworks (MOFs)and/or coordination polymers.These materials not only have interesting molecular structures and fascinating variety of topologies,but also have potential applications in gas adsorption and separation,luminescence,non-linear optics,phase transformation,magnetism and heterogeneous catal-ysis [1–5].However,the controllable synthesis of MOFs with desired architectures is still a great challenge because there are many chemical and physical factors that play important roles in the assembly process,such as the molecular structures of the ligands,nature of the metal ions,reaction temperature,pH value,solvent,and supra-molecular interactions such as hydrogen bonding and p –p stacking interactions [6–9].Therefore,a great deal of effort is required to extend our knowledge of structural design and to establish proper synthetic strategies that lead to the desired species with predictable structures and properties.Generally,the selection of a suitable ligand is the key step in engendering MOFs with desirable dimension.So far,a variety of coordination polymers with different bridging ligands have been synthesized.In particular,bi-or multi-dentate flexible bridging ligands containing N-or O-donors have been comprehensively used to bind metal centers to generate one-,two-and three-dimensional infinite metal–organic frameworks [10–15].Bis(benzimidazole)-containing flexible ligands on para -,meta -and ortho -xylene as a bridging spacer moiety have been well-used in crystal engi-neering and are of particular interest in coordination chem-istry [16–19],since they are effective bridging ligands.The flexible nature of –(Ph-CH 2)–spacers allows the ligand to bend and rotate when it coordinates to metal centers so as to conform to the coordination geometries of metal ions [20–22].In contrast,the most outstanding compound of benzimidazole derivatives are 5,6–dimethylbenzimidazole,which serves as an axial ligand for cobalt in vitamin B 12[23].To the best ofElectronic supplementary material The online version of this article (doi:10.1007/s10904-013-9825-x )contains supplementary material,which is available to authorized users.S.L.Xiao ÁL.Qin ÁC.H.He ÁX.Du ÁG.H.Cui (&)College of Chemical Engineering,Hebei United University,46West Xinhua Road,Tangshan 063009,Hebei,People’s Republic of China e-mail:tscghua@123J Inorg Organomet Polym (2013)23:771–778DOI 10.1007/s10904-013-9825-xour knowledge,coordination polymers based onflexible bis(5,6–dimethylbenzimidazole)ligands have been scarcely studied[24–26].In this paper,we have embarked on the synthesis of twoflexible bis-benzimidazole-based ligands, 1,4-bis(5,6-dimethylbenzimidazole-1-ylmethyl)benzene and 1,2-bis(5,6-dimethylbenzimidazole-1-ylmethyl)benzene.We used these ligands to react with a Cd(II)salt under hydro-thermal conditions;and,a2D[3,6]flat topology, {[Cd(L1)(chdc)]Á(CH3CN)Á(H2O)2}n(1),and a1D loop-like chain structure,[Cd(L2)Cl2]n(2),were obtained and characterized.2Experimental2.1Materials and Physical MeasurementsAll chemicals used were analytical reagents and commer-cially purchased(Sinopharm Chemical Reagent Co,Ltd).The hydrothermal syntheses were performed in Teflon-lined stainless steel autoclaves.Ligands L1and L2were prepared according to the literature[27](Scheme1).IR spectra were obtained with a Bruker Tensor27FT-IR spectrometer using KBr pellets in the4,000–400cm-1range.Elemental analyses for C,H and N were carried out with a Vario EL III elemental analyzer.The TG measurements were carried out with a NETZSCH TG209thermal analyzer from room temperature to800°C in a nitrogen atmosphere with a heating rate of 10°C min-1.X-ray powder diffraction(XRPD)was recor-ded on a Rigaku D/Max-2500diffractometer at40kV, 100mA for a CuK a tube(k=1.54178A˚).The photolumi-nescence spectra for compounds samples were determined with a Cary Eclipsefluorescence spectrophotometer.UV-Vis spectra of1,2and ligands L1and L2were obtained with an Hitachi UV-3010spectrophotometer in DMF solution (2.0910-5mol dm3)at298K.2.2Crystallographic Data Collection and Refinement The single crystal X-ray data of coordination polymers1 and2were collected using MoK a X-ray radiation with a Bruker SMART1000CCD diffractometer at298K.A single crystal suitable for X-ray diffraction was mounted on a glassfiber.The structure was solved by direct methods and refined by full-matrix least-squares based on F2using SHELXS-97and SHELXL-97[28].The non-hydrogen atoms were refined anisotropically and the H-atoms were geometrically included in thefinal refinement.Thefinal cycle of full-matrix least-squares refinement is based on observed reflections and variable parameters.In the struc-ture of1,the two lattice water and one CH3CN molecules are disordered.Thus,this structure was refined by the SQUEEZE routine of the PLATON program[29].The void solvent accessible volume of1is595.4A˚3and the percent of per unit cell volume is17.4%.Selected crystallographic and experimental details and also interatomic distances are summarized in Tables1and2.2.3Synthesis of{[Cd(L1)(chdc)]Á(CH3CN)Á(H2O)2}n(1)A mixture of Cd(NO3)2.4H2O(23.6mg,0.1mmol),L1(39.5mg,0.1mmol),H2chdc(34.4mg,0.1mmol),CH3 CN(1mL),and H2O(4mL)was sealed in a25mL Teflon-lined stainless vessel and heated to140°C for3-d under autogenous pressure,and then cooled to room tem-perature at a cooling rate of5°C h.The slightly yellow block-shaped crystal of1was obtained byfiltration.1was washed with distilled water and dried in the air;yield, 50.4%[based on Cd(NO3)2.4H2O].Anal.Calc.for C36H43CdN5O6(Mr=755.22)(%):C,57.2;H,5.7;N, 9.3.Found:C,56.9;H,5.6;N,9.1.FT-IR(KBr pellets, cm-1):3,380s,3,130m,2,939m,1,630w,1,539s,1,419s, 1,210m,1,060m,856s,630w,470m.2.4Synthesis of[Cd(L2)Cl2]n(2)By employing the above described procedure with a mixture of CdCl2(18.3mg,0.1mmol),L2(39.5mg,0.1mmol), ethanol(4mL),and H2O(6mL),colorless block-shaped crystals of2were obtained.2wasfiltered,washed with distilled water and dried in the air;yield,72.4%(based on CdCl2).Anal.Calc.for C26H26CdCl2N4(Mr=578.06)(%): C,54.0;H,4.5;N,9.7.Found:C,53.8;H,4.4;N,9.6.FT-IR123(KBr pellets,cm-1):3,088m,2,934m,1,490s,1,218m, 1,059w,863m,762m,622w,615w,432w.3Results and Discussion3.1IR and UV Absorption SpectraIn the FTIR spectra of1and2,the bands at1,539,1,210 and1,060cm-1for1and1,490,1,218,1,059cm-1for2 are assigned to benzimidazolyl ring vibrations.In1,a strong band at3,380cm-1is assigned to m OH stretching vibrations of the lattice water molecules.The broadness of this band suggests the H-bonding.The C=N stretching vibration of the benzimidazolyl group(1,539cm-1)is blue-shifted by48cm-1relative to free the free ligand (1,491cm-1),and is consistent with coordination of L1to Cd(II).Furthermore,the carboxylate group asymmetric and symmetric stretching vibrations are observed at1,720and 1,410cm-1,respectively,for free1,4-cyclohexanecarbox-ylic acid,while in1these bands occur at1,630and 1,419cm-1,respectively.The separation,D m[m as(COO)-m s(COO)],is111cm-1and indicates the presence of che-lating coordination of the carboxylic groups[30].The absorption spectra of the ligands1and2in DMF solution were measured at room temperature(Figure S1–S2,Supporting Information).The strong bands at266,282,291nm (for L1),265,283,291nm(for complex1),282,291nm(for L2),and264,285,291nm(for complex2)are ascribed to the p?p*transition within the benzimidazolyl rings.Table1Crystallographic data and structure refinement parameters for1and2Crystal data12Formula C36H43CdN5O6C26H26CdCl2N4 Formula weight755.22577.81 Crystal system Monoclinic Triclinic Space group C2/m P-1a(A˚)21.074(2)10.8341(15)b(A˚)16.5844(17)10.8631(15)c(A˚)11.4834(12)12.1669(17)a/deg9064.502(2)b/deg127.2760(10)85.092(2)c/deg9071.087(2)V(A˚3)3,430.2(6)1,220.3(3)Z42q calcd(g cm-3) 1.311 1.573l,mm-10.677 1.136F(000)1,392584 Collected reflection15,92610,219 Independent reflection4,0714,806 Goodness-of-fit on F20.9370.874R(int)0.07070.0310R1,wR2(I[2r(I))0.0421,0.08130.0337,0.0722 Largest residuals,e A˚-30.421/-0.5280.423/-0.393Table2Selected bond lengths(A˚)and angles(°)for1and2 Complex1Bond lengths(A˚)Cd1–N1B 2.278(2) Cd1–O3A 2.238(4) Cd1–N1 2.278(2) Cd1–O1 2.429(2) Cd1–O1B 2.429(2) Cd1–O2A 2.455(4) Bond angles(°)O3A–Cd1–N1132.81(16) O3A–Cd1–N1B110.39(17) N1–Cd1–N1B104.12(13) O3A–Cd1–O186.83(14) N1–Cd1–O1128.05(8)N1B–Cd1–O184.97(8)O3A–Cd1-O1B97.60(14) N1–Cd1–O1B84.97(8)N1B–Cd1–O1B128.05(8)O1–Cd1–O1B53.03(11) O3A–Cd1–O2A51.73(15) N1–Cd1–O2A94.95(8)N1B–Cd1–O2A94.95(8)O1–Cd1–O2A135.78(10) O1B–Cd1–O2A135.78(10) Complex2Bond lengths(A˚)Cd1–N3 2.261(3) Cd1–N1 2.357(3) Cd1–Cl2 2.4432(10) Cd1–Cl1 2.4769(9) Cd1–Cl1A 2.8101(9) Bond angles(°)N3–Cd1–N198.53(10) N3–Cd1–Cl2109.29(8)N1–Cd1–Cl295.94(7)N3–Cd1–Cl1111.10(7)N1–Cd1–Cl188.67(7)Cl2–Cd1–Cl1138.13(4)N3–Cd1–Cl1A91.62(7)N1–Cd1–Cl1A167.18(7)Cl2–Cd1–Cl1A87.99(3)Cl1–Cd1–Cl1A80.34(3) Symmetry codes1,A:-x?1,y,-z?1,B:x,-y?1,z;2,A: -x?1,-y?1,-z?21233.2Crystal Structure of 1and 2The structure of polymer 1was determined by single X-ray crystal diffraction.The ORTEP diagram of the coordination environment of Cd(II)atom is depicted in Fig.1a.The structural motif of 1consists of one 6-coordinated Cd(II)ion that located in the mirror plane of the C2/m space group,one-half a L1ligand,and one-half a chdc diatomic anion possessing chelating bis-bidentate carboxylate groups.The crystallographically independent Cd(II)center lies in a dis-torted octahedral coordination environment and is completed by two N-donors (N1and N1B)of two L1ligands and four O-donors (O1,O1B,O2A,and O3A,symmetry codes:A =-x ?1,y,-z ?1,B =x,-y ?1,z )from two chelating carboxylate groups of distinct chdc ligands.The atoms O1,O3A,O2A,N1compose the equatorial positions and N1B,O1B hold the axial positions.The Cd–O bondlengths range from 2.238(4)to 2.455(4)A˚,while the Cd–N1and Cd–N1B bond lengths are both 2.278(2)A˚,all lying within the reasonable bonding range as estimated from crystallographic determination (Table 2).The O–Cd–O or O–Cd–N or N–Cd–N bond angles are far from the typical octahedral 90°or 180°;they range from 51.73°to 135.78°for O–Cd–O,84.97°to 132.81°for O–Cd–N and 104.12°forFig.1a ORTEP drawing of 1with thermal ellipsoids drawn at 30%probability and the coordination environment of Cd(II)atom.The hydrogen atoms are omitted for clarity (Symmetry codes:A =-x ?1,y,-z ?1,B =x,-y ?1,z).b 2D flat structure of 1.c 2D (6,3)flat network of 1.d 2D supramolecular network of 1stabilized by p …p stacking interactions123N–Cd–N angles.Thus,as a node,the adjacent Cd(II)-atoms are infinitely linked by L1ligands to generate a zigzag chainwith shorter separations of Cd •••Cd being 14.8789(13)A˚and the angle of Cd–Cd–Cd being 67.74°.The zigzag chains are further bridged by chdc ligands,which adopt a bis-bidentate l 2-g 4bridging mode to connect two Cd(II)-atoms to form an 18-membered ring with the nearest Cd •••Cddistance between the zigzag chains of 7.8343(8)A˚(Fig.1b).The L1ligands exhibit a trans form with two coplanar benzimidazole rings from same L1ligand;the chdc ligands possess a cis form and the two hexamethylene rings from two chdc diatomic anions within one 18-membered annulus are parallel.Therefore,each 6-coordinated Cd(II)center is connected by two L1and two chdc ligands to form a 2D flat topography with honeycomb like 63topology (Fig.1c).In addition,the strong p –p stacking interactions between the benzene and imidazole rings from different L1ligands make the flat topologies more stable and result in self-assembly to a supramolecular structure with a centroid-to-centroid dis-tance of 3.65(7)A˚,slipping angles b or c of 16.12o or 16.62o ,and an average d p –p distance of 3.5034(12)A˚(Fig.1d).The X-ray diffraction study of 2reveals an asymmetric unit consisting of one Cd(II)center,one L2ligand,one terminal chlorine anion and two l 2bridging chlorine anions,which sit on inversion centers in triclinic P -1.Cd1is located in the center of a roughly square-pyramidal geometry (s factor,0.48[31]),which is formed by three Cl-atoms and two N-atoms from two bridging L2ligands (Fig.2a).The four atoms,Cl1,Cl2,Cl1A and N1,form a tapered bottom and the N-atom (N3)is located in the conic node.The distance from the conic node to the taperedbottom is 2.257A˚.The Cd–Cl bond lengths vary from 2.4432(10)to 2.8101(9)A˚,the Cd–N bond lengths vary from 2.261(3)to 2.357(3)A˚and the coordination bond angles range from 80.34(3)°to 167.18(7)°(Table 2).Complex 2displays an interesting infinite 1D loop-like chain structure composed of two kinds of rings;i.e.,a smaller 4-membered ring and the larger 24-membered ring (Fig.2b).The smaller ring is formed by twochlorineFig.2a ORTEP drawing of 2with thermal ellipsoids drawn at 30%probability and the coordination environment of Cd(II)atom.The hydrogen atoms are omitted for clarity.(Symmetry codes:A =-x ?1,-y ?1,-z ?2).b 1D infinite looped-like structure of 2.c 2D supramolecular structure of 2formed by p …p stacking interactions123anions bridging two Cd(II)ions with a CdÁÁÁCd distance is 4.0455(7)A˚;the larger ring is made up of two L2mole-cules bridging two Cd(II)ions with a CdÁÁÁCd distance is 15.834(2)A˚.Each L2exhibits a trans conformation with the dihedral angle between the two benzimidazole planes of81.259°.The above two kinds of rings are connected alternately via Cd(II)ions to form an infinite1D chain.Moreover,the adjacent looped chains are nearly parallel with a dihedral angle of1.267(4)°,which results in for-mation of strong p–p stacking interactions between benz-imidazole rings of distinct L2ligands.Thus,the looped chains are expanded to a2D supramolecular network by p–p stacking interactions(Fig.2c),with a center-to-center distance of3.541(2)A˚,an inter-planar angle a of1.27(2)°, slipping angles b or c of14.67o or13.92°and an average d p–p distance of3.431(4)A˚.In addition,there appears to be C–HÁÁÁCl hydrogen bonding interactions between benzyl C-atoms and Cl-atoms,which stabilize the2D supramo-lecular structure.The distance of H20AÁÁÁCl2is2.60(4)A˚and the bond angel for C20–H20AÁÁÁCl2is167°.Cd(II)coordination polymers based on the L1,L2or similar bis(benzimidazole)derivatives and polycarboxylates have been scarcely reported.Hou and co workers[32]prepared four Cd(II)-ferrocenesuccinate(FcCOC2H4COO)coordination compounds with the formulas[Cd(g2-FcCOC2H4COO)2 (pbbbm)]2,[Cd(g2-FcCOC2H4COO)(pbbbm)Cl]2,[Cd(g2-FcCOC2H4COO)-(pbbbm)I]2and{[Cd(g2-FcCOC2-H4 COO)(mbbbm)Cl].(H2O)2.75}n[pbbbm=1,4-bis(benzimid-azole-1-ylmethyl)benzene),mbbbm=1,3-bis(benzimidazole -1-ylmethyl)benzene)].The former three complexes are dimers and bridged by pbbbm and the last complex has chain-like structure bridged by chlorine anions and adjacent mbbbm ligand.Furthermore,Wang et al.reported two3D Cd(II) coordination polymers:[Cd3(bbbm)3(SIP)2(H2O)4].2H2O and [Cd(bbbm)(NIP)](bbbm=1,1-(1,4-butanediyl)bis-1H-benz-imidazole,H3SIP=5-sulfoisophthalic acid and H2NIP=5-nitroisophthalic acid)[33].In the[Cd3(bbbm)3(SIP)2(H2O)4]. 2H2O polymer,Cd(II)ions are connected by SIP anions to form 2D layers containing a12-membered macrocycle,which is further connected by the bbbm linkers to form a3D(3,3,4,4,4)-connected framework with a(3.6.73.8)(3.6.7)(3.62.73)(6.72) (6.74.10)topology.The complex,[Cd(bbbm)(NIP)],displays a 3D8-connected framework with a(424.64)topology connected by bbbm ligands and NIP anions.The complexes reported here have diverse structures:1displays a2D(6,3)flat network and2 exhibits a1D loop-like chain.This implies that different car-boxylates and anions play an important role in the assembly and structure of the title complexes.3.3Thermal PropertiesTo investigate the thermal stability of1and2,TGA were performed(Figure S3and Figure S4,Supporting Information).The decomposition of1occurs in two dis-tinct stages.Thefirst weight loss of7.9%from82.2to 202°C corresponds to the removal of water and acetoni-trile which are included in the crystal lattice(calcd.7.81%).The second mass loss occurs from237to502°C was75.6%and is assigned to the decomposition of the organic ligands,L1and chdc(calcd.75.7%).A residue of 16.4%(calcd.16.5%)suggests CdO asfinal decomposi-tion plex2is thermally stable to404°C. Then2rapidly loses weight from405to577°C,which corresponds to the decomposition of L2(observed67.9%, calcd.68.3%).The remaining mass(32.1%)is consistent with a CdCl2residue(calcd.31.7%).3.4XRPD ResultsThe powder XRPD pattern and simulated pattern of1and2 are depicted in Fig.3a–b.The measured and simulated powder patterns are in good agreement with the X-ray single crystal data,indicating phase purities of the samples. The difference in reflection intensities between the exper-imental and simulated patterns is due to the different ori-entation of the crystals in the powdered samples.1233.5Photoluminescence PropertiesCompounds of d 10metals exhibit some interesting photolu-minescent properties [34].The solid-state luminescence spectra of complexes 1and 2(Fig.4a,b)together with corresponding free ligands were investigated at room tem-perature under the same conditions.The free ligands exhibit strong fluorescence at k max =316nm (L1),346nm (L2)upon excitation at 298nm.The emission bands of the ligands are assigned to p ?p *transition [24–26].The emission spectra of the title complexes display photoluminescence with emission maxima at 402nm for 1,and 347nm for 2on excitation at pared to the emission of corre-sponding ligands,a red shift of 86nm for 1and 1nm for 2are observed.This suggests that the photoluminescence of the complexes originates mainly from the free ligands and are assigned to the p ?p *transition of the coordinated ligands since the Cd 2?ion is difficult to oxidize or reduce due to its d 10configuration [35–37].4ConclusionIn summary,two new Cd(II)coordination polymers based on flexible bis(5,6-dimethylbenzi-midazol)benzene,{[Cd(L1)(chdc)]Á(CH 3CN)Á(H 2O)2}n (1)and [Cd(L2)Cl 2]n (2),have been obtained and characterized.Structural analysis showed that 1displays a 2D (6,3)flat network linked by L1and chdc ligands,whereas 2has an infinite loop-like chain connected by L2ligands and chlorine atoms,then extended to 2D supramolecular structure by strong intermolecular p –p stacking interactions.This work presents that the different anions (or carboxylate anion)and the flexibility of ligands may exert a crucial influence on the formation of resulting diverse structures and supramolecular architectures.5Supplementary MaterialCrystallographic data for the structural analysis have been deposited with the Cambridge Crystallographic Data Centre with CCDC No:897111and 897112contain the supple-mentary crystallographic data for 1and 2,respectively.Copies of this information may be obtained free of charge on application to CCDC,12Union Road,Cambridge CB21EZ,UK (Fax:?44-1223-336033;E-mail:deposit@ or on the web ).The absorp-tion spectra of the ligands and complexes and the TG curves of 1and 2(Fig.S1–S4)are available from the corresponding author at tscghua@References1.R.Custelcean,Chem.Soc.Rev.39,3675(2010)2.B.Moulton,M.J.Zaworotko,Chem.Rev.101,1629(2001)3.K.K.Tanabe,S.M.Cohen,Chem.Soc.Rev.40,498(2011)4.M.Pan,X.M.Lin,G.B.Li,C.Y.Su,Coord.Chem.Rev.255,1921(2011)5.F.A.A.Paz,J.Klinowski,S.M.F.Vilela,J.P.C.Tome´,J.A.S.Cavaleiro,J.Rocha,Chem.Soc.Rev.41,1088(2012)6.D.S.Chowdhuri,M.Bera,A.Rana,D.Hazari,S.K.Jana,E.Zangrando,S.Dalai,anomet.Polym.Mater.22,963(2012)7.M.S.Y.Parast,A.Morsali,anomet.Polym.Mater 22,998(2012)8.L.Yan,C.B.Li,D.S.Zhu,L.Xu,anomet.Polym Mater.22,235(2012)9.Y.Ding,Z.B.Fu,X.L.Hu,C.F.Xia,anomet.Polym.Mater.20,642(2010)10.J.C.Geng,G.H.Cui,C.H.Jiao,T.F.Liu,C.H.He,J.Chin.Chem.Soc.59,704(2012)11.X.L.Wang,Y.F.Bi,H.Y.Lin,G.C.Liu,Cryst.Growth Des.7,1086(2007)12.X.L.Wang,H.Y.Lin,G.C.Liu,B.K.Chen,Y.F.Bi,Chem.Res.Chin.Univ.24,129(2008)13.X.L.Wang,J.X.Zhang,G.C.Liu,H.Y.Lin,Y.Q.Chen,Z.H.Kang,Inorg.Chim.Acta 368,207(2011)14.X.L.Wang,S.Yang,G.C.Liu,J.X.Zhang,H.Y.Lin,A.X.Tian,Inorg.Chim.Acta 375,70(2011)15.G.H.Cui,C.H.He,C.H.Jiao,J.C.Geng,V.A.Blatovb,mun.14,4210(2012)16.C.H Jiao,J.C.Geng,C.H.He,G.H.Cui,J.Mol.Struct.1020,134(2012)Fig.4Fluorescence emission spectra of a ligand L1and complex 1.b Ligand L2and complex12317.C.H.Jiao,C.H.He,J.C.Geng,G.H.Cui,J.Coord.Chem.65,2852(2012)18.J.C.Geng,C.H.Jiao,J.M.Hao,G.H.Cui,Z.Naturforsch.67b,791(2012)19.Z.Zhang,M.Pi,T.Wang,C.M.Jin,J.Mol.Struct.992,111(2011)20.J.Yao,Z.D.Lu,Y.Z.Li,J.G.Lin,X.Y.Duan,S.Gao,Q.J.Meng,C.S.Lu,mun.10,1379(2008)21.S.R.Zhu,H.Zhang,Y.M.Zhao,M.Shao,Z.X.Wang,M.X.Li,J.Mol.Struct.892,420(2008)n,S.L.Li,J.S.Qin,D.Y.Du,X.L.Wang,Z.M.Su,Q.Fu,Inorg.Chem.47,10600(2008)23.S.S.Marwaha,R.P.Sethi,J.F.Kennedy,Enzyme Microb.Technol.5,361(1983)24.C.H.He,C.H.Jiao,J.C.Geng,G.H.Cui,J.Coord.Chem.65,2294(2012)25.J.C.Geng,L.Qin,C.H.He,G.H.Cui,Transition Met.Chem.37,579(2012)26.S.L.Xiao,X.Du,L.Qin,C.H.He,G.H.Cui,ano-met.Polym.Mater.22,1384(2012)27.C.B.Aakeroy,J.Desper,E.Elisabeth,B.A.Helfrich,B.Levin,J.F.Urbina,Z.Kristallogr.220,325(2005)28.G.M.Sheldrick,Acta Crystallogr.A64,112(2008)29.A.L.Spek,A.Platon,Multipurpose Crystallographic Tool(Utr-echt University,Utrecht,2000)30.G.B.Deacon,R.J.Phillips,Coord.Chem.Rev.33,227(1980)31.A.W.Addison,T.N.Rao,J.Chem.Soc.,Dalton Trans.1349(1984)32.J.P.Li,L.K.Li,H.W.Hou,Y.T.Fan,anomet.Chem.694,1359(2009)33.X.L.Wang,L.L.Hou,J.W.Zhang,J.X.Zhang,G.C.Liu,S.Yang,mun.14,3936(2012)34.Q.Y.Liu,Y.L.Wang,L.Xu,Eur.J.Inorg.Chem.23,4843(2006)35.Y.B.Dong,H.Y.Wang,J.P.Ma,D.Z.Shen,R.Q.Huang,Inorg.Chem.44,4679(2005)36.J.L.Yin,Y.L.Feng,n,Inorg.Chim.Acta362,3769(2009)37.S.Geranmayeh,A.Abbasi,M.Y.Skripkin,A.Badiei,Polyhedron45,204(2012)123。