Sb-NQR probe for superconducting property in the Pr-based filled skutterudite compound PrRu
NVIDIA Jetson AGX Orin系列产品数据手册说明书
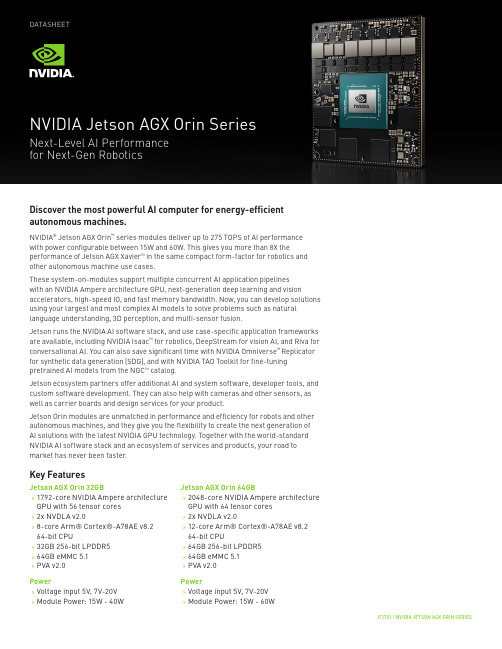
P3701 | NVIDIA JETSON AGX ORIN SERIESDATASHEETDiscover the most powerful AI computer for energy-efficient autonomous machines.NVIDIA ® Jetson AGX Orin ™ series modules deliver up to 275 TOPS of AI performance with power configurable between 15W and 60W. This gives you more than 8X theperformance of Jetson AGX Xavier ™ in the same compact form-factor for robotics and other autonomous machine use cases.These system-on-modules support multiple concurrent AI application pipelines with an NVIDIA Ampere architecture GPU, next-generation deep learning and visionaccelerators, high-speed IO, and fast memory bandwidth. Now, you can develop solutions using your largest and most complex AI models to solve problems such as natural language understanding, 3D perception, and multi-sensor fusion.Jetson runs the NVIDIA AI software stack, and use case-specific application frameworks are available, including NVIDIA Isaac ™ for robotics, DeepStream for vision AI, and Riva for conversational AI. You can also save significant time with NVIDIA Omniverse ™ Replicator for synthetic data generation (SDG), and with NVIDIA TAO Toolkit for fine-tuning pretrained AI models from the NGC ™ catalog.Jetson ecosystem partners offer additional AI and system software, developer tools, and custom software development. They can also help with cameras and other sensors, as well as carrier boards and design services for your product.Jetson Orin modules are unmatched in performance and efficiency for robots and other autonomous machines, and they give you the flexibility to create the next generation of AI solutions with the latest NVIDIA GPU technology. Together with the world-standard NVIDIA AI software stack and an ecosystem of services and products, your road to market has never been faster.Jetson AGX Orin 32GB>1792-core NVIDIA Ampere architecture GPU with 56 tensor cores >2x NVDLA v2.0>8-core Arm® Cortex®-A78AE v8.2 64-bit CPU>32GB 256-bit LPDDR5 >64GB eMMC 5.1 >PVA v2.0Power>Voltage input 5V, 7V-20V >Module Power: 15W - 40WKey FeaturesJetson AGX Orin 64GB>2048-core NVIDIA Ampere architecture GPU with 64 tensor cores >2x NVDLA v2.0>12-core Arm® Cortex®-A78AE v8.2 64-bit CPU>64GB 256-bit LPDDR5 >64GB eMMC 5.1 >PVA v2.0Power>Voltage input 5V, 7V-20V >Module Power: 15W - 60WNVIDIA JETSON AGX ORIN SERIES MODULES TECHNICAL SPECIFICATIONS* Virtual channel-related camera information for Jetson AGX Orin is not final and subject to change.Refer to the Software Features section of the latest NVIDIA Jetson Linux Developer Guide for a list of supportedfeatures.。
低场核磁共振测量孔径原理
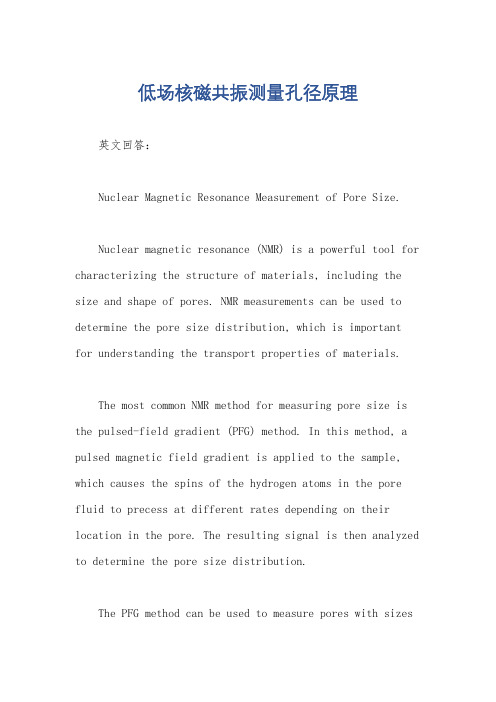
低场核磁共振测量孔径原理英文回答:Nuclear Magnetic Resonance Measurement of Pore Size.Nuclear magnetic resonance (NMR) is a powerful tool for characterizing the structure of materials, including the size and shape of pores. NMR measurements can be used to determine the pore size distribution, which is importantfor understanding the transport properties of materials.The most common NMR method for measuring pore size is the pulsed-field gradient (PFG) method. In this method, a pulsed magnetic field gradient is applied to the sample, which causes the spins of the hydrogen atoms in the pore fluid to precess at different rates depending on their location in the pore. The resulting signal is then analyzed to determine the pore size distribution.The PFG method can be used to measure pores with sizesranging from a few nanometers to several microns. The accuracy of the measurement depends on the strength of the magnetic field gradient and the duration of the pulse.Another NMR method for measuring pore size is the relaxation method. In this method, the relaxation times of the hydrogen atoms in the pore fluid are measured. The relaxation times are affected by the size and shape of the pores, and can therefore be used to determine the pore size distribution.The relaxation method is less accurate than the PFG method, but it can be used to measure pores with sizes ranging from a few nanometers to several hundred microns.中文回答:核磁共振测孔原理。
Monotonic d-wave Superconducting Gap in Optimally-Doped Bi$_2$Sr$_{1.6}$La$_{0.4}$CuO$_6$ S
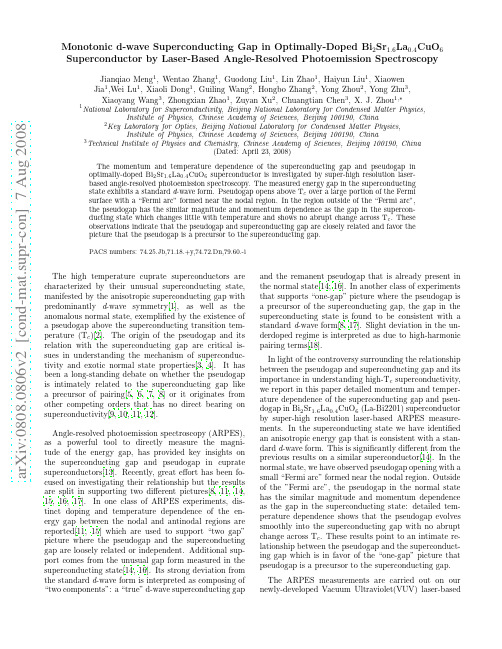
a r X i v :0808.0806v 2 [c o n d -m a t .s u p r -c o n ] 7 A u g 2008Monotonic d-wave Superconducting Gap in Optimally-Doped Bi 2Sr 1.6La 0.4CuO 6Superconductor by Laser-Based Angle-Resolved Photoemission SpectroscopyJianqiao Meng 1,Wentao Zhang 1,Guodong Liu 1,Lin Zhao 1,Haiyun Liu 1,Xiaowen Jia 1,Wei Lu 1,Xiaoli Dong 1,Guiling Wang 2,Hongbo Zhang 2,Yong Zhou 2,Yong Zhu 3,Xiaoyang Wang 3,Zhongxian Zhao 1,Zuyan Xu 2,Chuangtian Chen 3,X.J.Zhou 1,∗1National Laboratory for Superconductivity,Beijing National Laboratory for Condensed Matter Physics,Institute of Physics,Chinese Academy of Sciences,Beijing 100190,China2Key Laboratory for Optics,Beijing National Laboratory for Condensed Matter Physics,Institute of Physics,Chinese Academy of Sciences,Beijing 100190,China3Technical Institute of Physics and Chemistry,Chinese Academy of Sciences,Beijing 100190,China(Dated:April 23,2008)The momentum and temperature dependence of the superconducting gap and pseudogap in optimally-doped Bi 2Sr 1.6La 0.4CuO 6superconductor is investigated by super-high resolution laser-based angle-resolved photoemission spectroscopy.The measured energy gap in the superconducting state exhibits a standard d -wave form.Pseudogap opens above T c over a large portion of the Fermi surface with a “Fermi arc”formed near the nodal region.In the region outside of the “Fermi arc”,the pseudogap has the similar magnitude and momentum dependence as the gap in the supercon-ducting state which changes little with temperature and shows no abrupt change across T c .These observations indicate that the pseudogap and superconducting gap are closely related and favor the picture that the pseudogap is a precursor to the superconducting gap.PACS numbers:74.25.Jb,71.18.+y,74.72.Dn,79.60.-iThe high temperature cuprate superconductors are characterized by their unusual superconducting state,manifested by the anisotropic superconducting gap with predominantly d -wave symmetry[1],as well as the anomalous normal state,exemplified by the existence of a pseudogap above the superconducting transition tem-perature (T c )[2].The origin of the pseudogap and its relation with the superconducting gap are critical is-sues in understanding the mechanism of superconduc-tivity and exotic normal state properties[3,4].It has been a long-standing debate on whether the pseudogap is intimately related to the superconducting gap like a precursor of pairing[5,6,7,8]or it originates from other competing orders that has no direct bearing on superconductivity[9,10,11,12].Angle-resolved photoemission spectroscopy (ARPES),as a powerful tool to directly measure the magni-tude of the energy gap,has provided key insights on the superconducting gap and pseudogap in cuprate superconductors[13].Recently,great effort has been fo-cused on investigating their relationship but the results are split in supporting two different pictures[8,11,14,15,16,17].In one class of ARPES experiments,dis-tinct doping and temperature dependence of the en-ergy gap between the nodal and antinodal regions are reported[11,15]which are used to support “two gap”picture where the pseudogap and the superconducting gap are loosely related or independent.Additional sup-port comes from the unusual gap form measured in the superconducting state[14,16].Its strong deviation from the standard d -wave form is interpreted as composing of “two components”:a “true”d-wave superconducting gapand the remanent pseudogap that is already present in the normal state[14,16].In another class of experiments that supports “one-gap”picture where the pseudogap is a precursor of the superconducting gap,the gap in the superconducting state is found to be consistent with a standard d -wave form[8,17].Slight deviation in the un-derdoped regime is interpreted as due to high-harmonic pairing terms[18].In light of the controversy surrounding the relationship between the pseudogap and superconducting gap and its importance in understanding high-T c superconductivity,we report in this paper detailed momentum and temper-ature dependence of the superconducting gap and pseu-dogap in Bi 2Sr 1.6La 0.4CuO 6(La-Bi2201)superconductor by super-high resolution laser-based ARPES measure-ments.In the superconducting state we have identified an anisotropic energy gap that is consistent with a stan-dard d -wave form.This is significantly different from the previous results on a similar superconductor[14].In the normal state,we have observed pseudogap opening with a small “Fermi arc”formed near the nodal region.Outside of the ”Fermi arc”,the pseudogap in the normal state has the similar magnitude and momentum dependence as the gap in the superconducting state:detailed tem-perature dependence shows that the pseudogap evolves smoothly into the superconducting gap with no abrupt change across T c .These results point to an intimate re-lationship between the pseudogap and the superconduct-ing gap which is in favor of the “one-gap”picture that pseudogap is a precursor to the superconducting gap.The ARPES measurements are carried out on our newly-developed Vacuum Ultraviolet(VUV)laser-based2E - EF (eV)E - EF (eV)1.00.50G (0,0)(p ,0)1510152025k xFIG.1:Fermi surface of the optimally-doped La-Bi2201(T c =32K)and corresponding photoemission spectra (EDCs)on the Fermi surface at various temperatures.(a).Spectral weight as a function of two-dimensional momentum (k x ,k y )integrated over [-5meV,5meV]energy window with respect to the Fermi level E F .The measured Fermi momenta are marked by red empty circles and labeled by numbers;(b).Original EDCs along the Fermi surface measured at 15K.The symmetrized EDCs along the Fermi surface are shown in (c)for 15K,(d)for 25K and (e and f)for 40K.The numbers on panels (b-f)corresponds to the Fermi momentum numbers in (a).angle-resolved photoemission system with advantages of super-high energy resolution,high momentum resolution,high photon flux and enhanced bulk sensitivity[19].The photon energy is 6.994eV with a bandwidth of 0.26meV and the energy resolution of the electron energy analyzer (Scienta R4000)was set at 0.5meV,giving rise to an overall energy resolution of 0.56meV.The angular res-olution is ∼0.3◦,corresponding to a momentum resolu-tion ∼0.004˚A −1at the photon energy of 6.994eV.The optimally doped Bi 2Sr 2−x La x CuO 6(La-Bi2201)(x=0.4,T c ∼32K,transition width ∼2K)single crystals were grown by the traveling solvent floating zone method[20].One advantage of choosing La-Bi2201system lies in its relatively low superconducting transition temperature that is desirable in investigating the normal state behav-ior with suppressed thermal broadening of photoemission spectra.The samples are cleaved in situ in vacuum with a base pressure better than 4×10−11Torr.Fig.1(a)shows the Fermi surface mapping of the op-timally doped La-Bi2201(T c =32K)measured at 15K.The low photon energy and high photon flux have made it possible to take dense sampling of the measurements in the momentum space.The photoemission spectra (En-ergy Distribution Curves,EDCs)along the Fermi surface are plotted in Fig.1(b).The EDCs near the nodal re-gion show sharp peaks that are similar to those observed in Bi2212[21].When the momentum moves away from the nodal region to the (0,π)antinodal region,the EDC peaks get weaker,but peak feature remains along the en-tire Fermi surface even for the one close to the antinodal region.The EDC peak position also shifts away from the Fermi level when the momentum moves from the nodal to the antinodal region,indicating a gap opening in the superconducting state.Note that the EDCs near the antinodal region do not show any feature near 40meV that was reported in a previous measurement[14].In order to extract the energy gap,we have sym-metrized the original EDCs with respect to the Fermi level,as shown in Fig.1c for the 15K measurements,and Fig.1d and Fig.1(e-f)for 25K and 40K,respec-tively.The symmetrization procedure not only provides an intuitive way in visualizing the energy gap,but also removes the effect of Fermi cutoffin photoemission spec-tra and provides a quantitative way in extracting the gap size[22].The symmetrized EDCs have been fitted using the general phenomenological form[22];the fitted curves are overlaid in Fig.1(c-f)and the extracted gap size is plotted in Fig.2.As shown in Fig.2,the gap in the superconducting state exhibits a clear anisotropic behavior that is consis-tent with a standard d -wave form ∆=∆0cos(2Φ)(or in a more strict sense,∆=∆0|cos (k x a )−cos (k y a )|/2form as shown in the inset of Fig.2)with a maximum energy gap ∆0=15.5meV.It is also interesting to note that the gap is nearly identical for the 15K and 25K measurements for such a T c =32K superconductor.These results are significantly different from a recent measurement where the gap in the superconducting state deviates strongly from the standard d -wave form with an antinodal gap at 40meV[14].An earlier measurement[23]gave an antin-3G a p S i z e (m e V )Angle F (degrees)FIG.2:Energy gap along the Fermi surface measured at 15K (solid circles),25K (empty circles)and 40K (empty squares)on the optimally-doped La-Bi2201(T c =32K).The solid red line is fitted from the measured data at 15K which gives ∆=15.5cos(2Φ).The Φangle is defined as shown in the bottom-right inset.The upper-right inset shows the gap size as a function of |cos (k x a )−cos (k y a )|/2at 15K and 25K.The pink line represents a fitted line with ∆=15.5|cos (k x a )−cos (k y a )|/2.odal gap at 10∼12meV which is close to our present mea-surement,but it also reported strong deviation from the standard d -wave form.While the non-d -wave energy gap can be interpreted ascomposed of two components in the previous measurement[14],our present results clearly in-dicate that the gap in the superconducting state is dom-inated by a d -wave component.In the normal state above T c =32K,the Fermi sur-face measured at 40K is still gapped over a large portion except for the section near the nodal region that shows a zero gap,as seen from the symmetrized EDCs (Fig.1e-f for 40K)and the extracted pseudo-gap (40K data in Fig.2).This is consistent with the “Fermi arc”picture observed in other high temperature superconductors[6,11,24].Note that the pseudogap out-side of the “Fermi arc”region shows similar magnitude and momentum dependence as the gap in the supercon-ducting state (Fig.2).Fig.3shows detailed temperature dependence of EDCs and the associated energy gap for two representa-tive momenta on the Fermi surface.Strong temperature dependence of the EDCs is observed for the Fermi mo-mentum A (Fig.3a).At high temperatures like 100K or above,the EDCs show a broad hump structure near -0.2eV with no observable peak near the Fermi level.Upon cooling,the high-energy -0.2eV broad hump shows little change with temperature,while a new structure emerges near the Fermi level and develops into a sharp “quasipar-ticle”peak in the superconducting state,giving rise to a peak-dip-hump structure in EDCs.This temperatureE - EF (eV)E - EF (eV)FIG.3:(a,b).Temperature dependence of representa-tive EDCs at two Fermi momenta on the Fermi surface in optimally-doped La-Bi2201.The location of the Fermi mo-menta is indicated in the inset.Detailed temperature depen-dence of the symmetrized EDCs for the Fermi momentum A are shown in (c)and for the Fermi momentum B in (d).The dashed lines in (c)and (d)serve as a guide to the eye.evolution and peak-dip-hump structure are reminiscent to that observed in other high temperature superconduc-tors like Bi2212[25].When moving towards the antin-odal region,as for the Fermi momentum B (Fig.3b),the EDCs qualitatively show similar behavior although the temperature effect gets much weaker.One can still see a weak peak developed at low temperatures,e.g.,13K,near the Fermi level.To examine the evolution of the energy gap with tem-perature,Fig.3c and 3d show symmetrized EDCs mea-sured at different temperatures for the Fermi momenta A and B,respectively.The gap size extracted by fit-ting the symmetrized EDCs with the general formula[22]are plotted in Fig. 4.For the Fermi momentum A,as seen from Fig.3c,signature of gap opening in the su-perconducting state persists above T c =32K,remaining obvious at 50K,getting less clear at 75K,and appear to disappear around 100K and above as evidenced by the appearance of a broad peak.The gap size below 50K (Fig.4)shows little change with temperature and no abrupt change is observed across T c .The data at 75K is hard to fit to get a reliable gap size,thus not included in Fig. 4.When the momentum moves closer to the antinodal region,as for the Fermi momentum B,simi-lar behaviors are observed,i.e.,below 50K,the gap size is nearly a constant without an abrupt change near T c .But in this case,different from the Fermi momentum A,4G a p S i z e (m e V )Temperature(K)FIG.4:Temperature dependence of the energy gap for two Fermi momenta A (empty squares)and B (empty circles)as indicated in insets of Fig.3(a)and (b),and also indicated in the up-right inset,for optimally-doped La-Bi2201.The dashed line indicates T c =32K.there is no broad peak recovered above 100K,probably indicating a higher pseudogap temperature.This is qual-itatively consistent with the transport[26]and NMR[27]measurements on the same material that give a pseudo-gap temperature between 100∼150K.From precise gap measurement,there are clear signa-tures that can distinct between “one-gap”and “two-gap”scenarios[4].In the “two-gap”picture where the pseudo-gap and superconducting gap are assumed independent,because the superconducting gap opens below T c in addi-tion to the pseudogap that already opens in the normal state and persists into the superconducting state,one would expect to observe two effects:(1).Deviation of the energy gap from a standard d -wave form in the super-conducting state with a possible break in the measured gap form[14];(2).Outside of the “Fermi arc”region,one should expect to see an increase in gap size in the superconducting state.Our observations of standard d -wave form in the superconducting state (Fig.2),similar magnitude and momentum dependence of the pseudogap and the gap in the superconducting state outside of the “Fermi arc”region (Fig.2),smooth evolution of the gap size across T c and no indication of gap size increase upon entering the superconducting state (Fig.4),are not com-patible with the expectations of the “two-gap”picture.They favor the “one-gap”picture where the pseudogap and superconducting gap are closely related and the pseu-dogap transforms into the superconducting gap across T c .Note that,although the region outside of the “Fermi arc”shows little change of the gap size with temperature (Fig.4),the EDCs exhibit strong temperature depen-dence with a “quasiparticle”peak developed in the su-perconducting state(Fig.3a and 3b)that can be related with the establishment of phase coherence[8,25].This suggests that the pseudogap region on the Fermi surface can sense the occurrence of superconductivity through acquiring phase coherence.In conclusion,from our precise measurements on the detailed momentum and temperature dependence of the energy gap in optimally doped La-Bi2201,we provide clear evidence to show that the pseudogap and super-conducting gap are intimately related.Our observations are in favor of the “one-gap”picture that the pseudogap is a precursor to the superconducting gap and supercon-ductivity is realized by establishing a phase coherence.We acknowledge helpful discussions with T.Xi-ang.This work is supported by the NSFC(10525417and 10734120),the MOST of China (973project No:2006CB601002,2006CB921302),and CAS (Projects IT-SNEM and 100-Talent).∗Corresponding author:XJZhou@[1]See,e.g.,C.C.Tsuei and J.R.Kirtley,Rev.Mod.Phys.72,969(2000).[2]T.Timusk and B.Statt,Rep.Prog.Phys.62,61(1999).[3]V.J.Emery and S.A.Kivelson,Nature (London)374,434(1995);X.G.Wen and P.A.Lee,Phys.Rev.Lett.76,503(1996);C.M.Varma,Phys.Rev.Lett.83,3538(1999);S.Chakravarty et al.,Phys.Rev.B 63,094503(2001);P.W.Anderson,Phys.Rev.Lett.96,017001(2006).[4]lis,Science 314,1888(2006).[5]Ch.Renner et al.,Phys.Rev.Lett.80,149(1998).[6]M.R.Norman et al.,Nature (London)392,157(1998).[7]Y.Y.Wang et al.,Phys.Rev.B 73,024510(2006).[8]A.Kanigel et al.,Phys.Rev.Lett.99,157001(2007).[9]G.Deytscher,Nature (London)397,410(1999).[10]M.Le.Tacon et al.,Nature Phys.2,537(2006).[11]K.Tanaka et al.,Scinece 314,1910(2006).[12]M.C.Boyer et al.,Nature Phys.3,802(2007).[13]A.Damascelli et al.,Rev.Mod.Phys.75,473(2003);J.C.Campuzano et al.,in The Physics of Superconductors,Vol.2,edited by K.H.Bennemann and J.B.Ketterson,(Springer,2004).[14]T.Kondo et al.,Phys.Rev.Lett.98,267004(2007).[15]W.S.Lee et al.,Nature (London)450,81(2007).[16]K.Terashima et al.,Phys.Rev.Lett.99,017003(2007).[17]M.Shi et al.,arXiv:cond-mat/0708.2333.[18]J.Mesot et al.,Phys.Rev.Lett.83,840(1999).[19]G.D Liu et al.,Rev.Sci.Instruments 79,023105(2008).[20]J.Q.Meng et al.,unpublished work.[21]W.T.Zhang et al.,arXiv:cond-mat/0801.2824.[22]M.R.Norman et al.,Phys.Rev.B 57,R11093(1998).[23]J.M.Harris et al.,Phys.Rev.Lett.79,143(1997).[24]A.Kanigel et al.,Nature Phys.2447(2006).[25]A.V.Fedorov et al.,Phys.Rev.Lett.82,2179(1999);D.L.Feng et al.,Science 289,277(2000);H.Ding et al.,Phys.Rev.Lett.87,227001(2001).[26]Y.Ando et al.,Phys.Rev.Lett.93,267001(2004).[27]G.-Q.Zheng et al.,Phys.Rev.Lett.94,047006(2005).。
Superconducting qubits II Decoherence

The transition from quantum to classical physics, now known as decoherence, has intrigued physicists since the formulation of quantum mechanics (Giulini et al., 1996; Leggett, 2002; Peres, 1993; Feynman and Vernon, 1963; Zurek, 1993). It has been put into the poignant Schr¨ odinger cat paradox (Schr¨ odinger, 1935) and was considered an open fundamental question for a long time.
and compare it to the corresponding classical mixture leading to the same expectation value of σz 1 1 0 ρmix = (2) 2 0 1 we can see that the von-Neumann entropy ρ = −kB Tr [ρ log ρ] rises from Spure = 0 to Smix = kB ln 2. Hence, decoherence taking ρpure to ρmix creates entropy and is irreversible. Quantum mechanics, on the other hand, is always reversible. It can be shown, that any isolated quantum system is described by the Liouville von-Neumann equation i¯ hρ ˙ = [H, ρ] (3)
Topological defects in triplet superconductors UPt$_{3}$, Sr$_{2}$RuO$_{4}$, etc
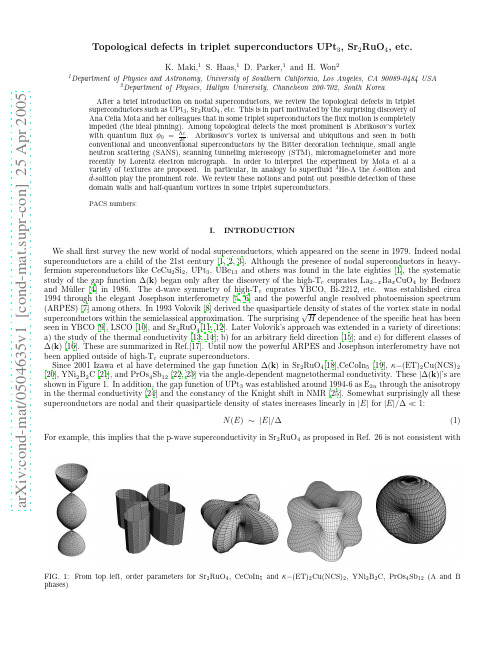
2
FIG. 2: Phase diagram of U1−xThxBe13 from Heffner et al [41].
the specific heat data [11]. Also as discussed elsewhere [27, 28], the two gap model is of little help in this matter. More recently the quasiparticle density of states in the vortex state in Sr2RuO4 has been reported [29]. Indeed the
observed quasiparticle density of states is very consistent with that predicted for an f-wave order parameter [30]. Also many of these superconductors are triplet: UPt3, Sr2RuO4, (TMTSF)2PF6, U1−xThxBe13, URu2Si2, PrOs4Sb12, UNi2Al3 and CePt3Si, for example.
After a brief introduction on nodal superconductors, we review the topological defects in triplet
superconductors such as UPt3, Sr2RuO4, etc. This is in part motivated by the surprising discovery of
Since 2001 Izawa et al have determined the gap function ∆(k) in Sr2RuO4[18],CeCoIn5 [19], κ−(ET)2Cu(NCS)2 [20], YNi2B2C [21], and PrOs4Sb12 [22, 23] via the angle-dependent magnetothermal conductivity. These |∆(k)|’s are shown in Figure 1. In addition, the gap function of UPt3 was established around 1994-6 as E2u through the anisotropy in the thermal conductivity [24] and the constancy of the Knight shift in NMR [25]. Somewhat surprisingly all these superconductors are nodal and their quasiparticle density of states increases linearly in |E| for |E|/∆ ≪ 1:
华为突破技术封锁自主研发芯片英语作文
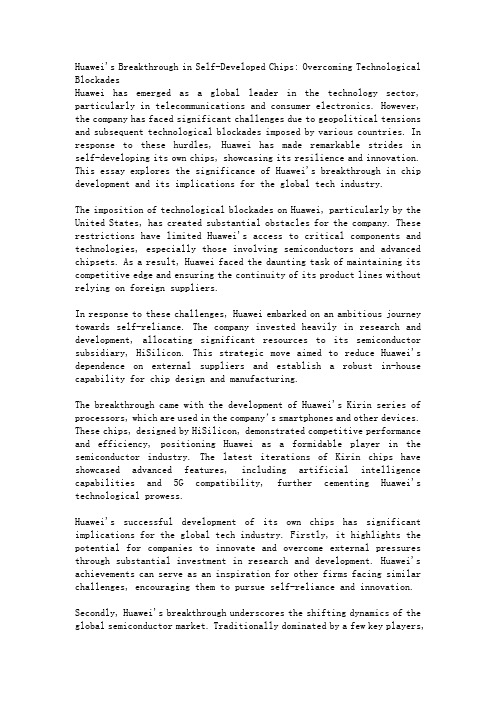
Huawei's Breakthrough in Self-Developed Chips: Overcoming Technological BlockadesHuawei has emerged as a global leader in the technology sector, particularly in telecommunications and consumer electronics. However, the company has faced significant challenges due to geopolitical tensions and subsequent technological blockades imposed by various countries. In response to these hurdles, Huawei has made remarkable strides in self-developing its own chips, showcasing its resilience and innovation. This essay explores the significance of Huawei's breakthrough in chip development and its implications for the global tech industry.The imposition of technological blockades on Huawei, particularly by the United States, has created substantial obstacles for the company. These restrictions have limited Huawei's access to critical components and technologies, especially those involving semiconductors and advanced chipsets. As a result, Huawei faced the daunting task of maintaining its competitive edge and ensuring the continuity of its product lines without relying on foreign suppliers.In response to these challenges, Huawei embarked on an ambitious journey towards self-reliance. The company invested heavily in research and development, allocating significant resources to its semiconductor subsidiary, HiSilicon. This strategic move aimed to reduce Huawei's dependence on external suppliers and establish a robust in-house capability for chip design and manufacturing.The breakthrough came with the development of Huawei's Kirin series of processors, which are used in the company’s smartphones and other devices. These chips, designed by HiSilicon, demonstrated competitive performance and efficiency, positioning Huawei as a formidable player in the semiconductor industry. The latest iterations of Kirin chips have showcased advanced features, including artificial intelligence capabilities and 5G compatibility, further cementing Huawei's technological prowess.Huawei's successful development of its own chips has significant implications for the global tech industry. Firstly, it highlights the potential for companies to innovate and overcome external pressures through substantial investment in research and development. Huawei's achievements can serve as an inspiration for other firms facing similar challenges, encouraging them to pursue self-reliance and innovation.Secondly, Huawei's breakthrough underscores the shifting dynamics of the global semiconductor market. Traditionally dominated by a few key players,the market is now witnessing the rise of new contenders from diverse regions. This increased competition can drive further innovation and potentially lead to more affordable and advanced technologies for consumers worldwide.Furthermore, Huawei's advancements in chip technology can contribute to the development of a more resilient global supply chain. By diversifying sources of critical components, the tech industry can reduce vulnerabilities and mitigate the risks associated with geopolitical tensions and trade restrictions.In conclusion, Huawei's breakthrough in self-developing chips represents a significant milestone in the face of technological blockades. Through substantial investment in research and development, the company has demonstrated its resilience and capability to innovate independently. This achievement not only strengthens Huawei's position in the global tech industry but also has broader implications for innovation, competition, and supply chain resilience. As Huawei continues to push the boundaries of technology, it serves as a powerful example of how challenges can be transformed into opportunities for growth and advancement.。
微芯片公司SyncServer S650高精确、安全和灵活的时间与频率标准说明书
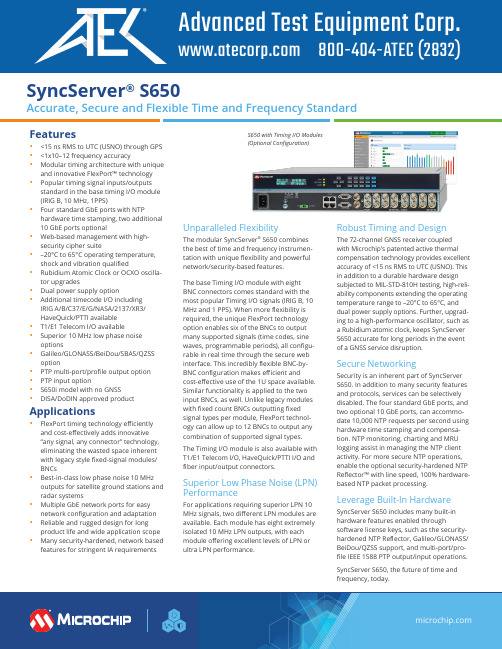
SyncServer® S650Accurate, Secure and Flexible Time and Frequency StandardFeatures•<15 ns RMS to UTC (USNO) through GPS •<1x10–12 frequency accuracy •Modular timing architecture with unique and innovative FlexPort™ technology •Popular timing signal inputs/outputs standard in the base timing I/O module(IRIG B, 10 MHz, 1PPS)•Four standard GbE ports with NTP hardware time stamping, two additional10 GbE ports optional•Web-based management with high-security cipher suite•–20°C to 65°C operating temperature, shock and vibration qualified •Rubidium Atomic Clock or OCXO oscilla-tor upgrades•Dual power supply option •Additional timecode I/O including IRIG A/B/C37/E/G/NASA/2137/XR3/HaveQuick/PTTI available•T1/E1 Telecom I/O available •Superior 10 MHz low phase noise options•Galileo/GLONASS/BeiDou/SBAS/QZSS option•PTP multi-port/profile output option •PTP input option•S650i model with no GNSS •DISA/DoDIN approved product Applications•FlexPort timing technology efficiently and cost-effectively adds innovative“any signal, any connector” technology,eliminating the wasted space inherentwith legacy style fixed-signal modules/BNCs•Best-in-class low phase noise 10 MHz outputs for satellite ground stations and radar systems•Multiple GbE network ports for easy network configuration and adaptation •Reliable and rugged design for long product life and wide application scope •Many security-hardened, network basedfeatures for stringent IA requirementsS650 with Timing I/O Modules(Optional Configuration)Unparalleled FlexibilityThe modular SyncServer® S650 combinesthe best of time and frequency instrumen-tation with unique flexibility and powerfulnetwork/security-based features.The base Timing I/O module with eightBNC connectors comes standard with themost popular Timing I/O signals (IRIG B, 10MHz and 1 PPS). When more flexibility isrequired, the unique FlexPort technologyoption enables six of the BNCs to outputmany supported signals (time codes, sinewaves, programmable periods), all configu-rable in real time through the secure webinterface. This incredibly flexible BNC-by-BNC configuration makes efficient andcost-effective use of the 1U space available.Similar functionality is applied to the twoinput BNCs, as well. Unlike legacy moduleswith fixed count BNCs outputting fixedsignal types per module, FlexPort technol-ogy can allow up to 12 BNCs to output anycombination of supported signal types.The Timing I/O module is also available withT1/E1 Telecom I/O, HaveQuick/PTTI I/O andfiber input/output connectors.Superior Low Phase Noise (LPN)PerformanceFor applications requiring superior LPN 10MHz signals, two different LPN modules areavailable. Each module has eight extremelyisolated 10 MHz LPN outputs, with eachmodule offering excellent levels of LPN orultra LPN performance.Robust Timing and DesignThe 72-channel GNSS receiver coupledwith Microchip's patented active thermalcompensation technology provides excellentaccuracy of <15 ns RMS to UTC (USNO). Thisin addition to a durable hardware designsubjected to MIL-STD-810H testing, high-reli-ability components extending the operatingtemperature range to –20°C to 65°C, anddual power supply options. Further, upgrad-ing to a high-performance oscillator, such asa Rubidium atomic clock, keeps SyncServerS650 accurate for long periods in the eventof a GNSS service disruption.Secure NetworkingSecurity is an inherent part of SyncServerS650. In addition to many security featuresand protocols, services can be selectivelydisabled. The four standard GbE ports, andtwo optional 10 GbE ports, can accommo-date 10,000 NTP requests per second usinghardware time stamping and compensa-tion. NTP monitoring, charting and MRUlogging assist in managing the NTP clientactivity. For more secure NTP operations,enable the optional security-hardened NTPReflector™ with line speed, 100% hardware-based NTP packet processing.Leverage Built-In HardwareSyncServer S650 includes many built-inhardware features enabled throughsoftware license keys, such as the security-hardened NTP Reflector, Galileo/GLONASS/BeiDou/QZSS support, and multi-port/pro-file IEEE 1588 PTP output/input operations.SyncServer S650, the future of time andfrequency, today. Four GbE Ports for Performance, Flexibility and SecurityThe S650 has four dedicated and isolated GbE Ethernet ports, each equipped with NTP hardware time stamping. These are connected to a high-speed microprocessor with microsecond-accurate timestamps to assure high-bandwidth NTP performance. This exceeds the need of servicing 10,000 NTP requests per second with no degrada-tion in time stamp accuracy.Multiple ports provide the flexibility to adapt to different network topologies as networks grow and change. An S650 can be the single time source to synchronize clients on different subnets and physi -cal networks. There is only one time reference, but it can appear as though there are four clocks available because each port is independent.NTP can be served on all four ports (six if 10 GbE ports are added). The highly secure web-based management interface is only available on port 1, so that administrators may choose to keep that IP address private and secure. Unique access control lists per port can govern server response to client requests for time.Intuitive, Secure and Easy-to-Use Web InterfaceThe modern web interface is the primary control interface of the S650. Once the keypad and display bring the unit online, complete status and control functions are easily found on the left navigation menu. A REST API also included.Standard Management Access SecurityAll of the expected network management protocols are standard in the S650. These include mandatory password access, HTTPS/SSL only (using the high-encryption cipher suite), SSH, access control lists, ser-vice termination, SNMPv2/v3, and NTP MD5 authentication. All traffic to the S650 CPU is bandwidth-limited for protection against DoSattacks. The local keypad on the server can be password-protected to prevent tampering.Security-Hardening OptionThe SyncServer S650 can be further hardened from both an NTP perspective and an authentication perspective through the Security Protocol License option that includes the security-hardened NTP Reflector.Operational hardening through the 360,000 NTP packet per second NTP Reflector with 100% hardware-based NTP packet processing also works with a CPU-protecting firewall by bandwidth limiting all non-NTP traffic. The Reflector also monitors packet flow for DoS detection and reporting, yet remains impervious to the level of network traffic as it operates at line speed.Authentication hardening is available for NTP client/server authenti-cation through the NTP Autokey function or user access authentica-tion through TACACS+, RADIUS and LDAP. Third party CA-signed X.509 certificates are installable for further hardening of manage -ment access and secure syslog operations. For more information about the Security Protocol License option, see the SyncServerOptions datasheet.The four GbE ports provide network configura -tion flexibility and enhanced security. Multiple isolated and synchronized time servers can also be configured. Two 10 GbE SFP+ ports can be added for NTP/PTP operations as well.At-a-glance dashboard presentation combined with logical organization andintuitive controls that make configuring the S650 easy.An entire drop-down menu in the S650 dedicated to security-related protocols.Unprecedented NTP AccuracyThe Stratum 1 level S650 derives nanosecond-accurate time directly from the atomic clocks aboard the GPS satellites. By using an integrated, 72-channel GNSS receiver, every visible satellite can be tracked and used to maintain accurate and reliable time. Even in urban canyon environments where direct satellite visibility can be limited, manually inputting the position can be sufficient to acquire accurate time from as few as one intermittent satellite.Ultra-High Performance NTPThe S650 can effortlessly support hundreds of thousands of network clients while maintaining microsecond-caliber NTP time stamp accuracy. NTP request throughput rates can exceed 10,000 requests/ second while maintaining NTP time stamp accuracy. NTP monitoring, charting and MRU logging assist in managing the NTP client activity. If the Security Protocol License option is enabled, the NTP Reflector can process over 360,000 NTP requests per second with 15-nanosecond caliber time stamp accuracy with the added benefit of security-hardening the network port.Superior Low Phase Noise PerformanceThe S650 can be optimized to provide the best possible low phase noise 10 MHz signals. Two LPN modules are available to choose from depending on the phase noise sensitivity of the user application. Each module has eight extremely isolated 10 MHz LPN outputs with each module offering excellent levels of LPN and Ultra LPN perfor-mance from the close in 1 Hz out to 100 kHz.Multi-Port/Profile IEEE 1588 PTP Grandmaster Applications demanding very precise time accuracy can require the IEEE 1588 precise time protocol (PTP). The S650 PTP Output License enables multi-port/profile PTP grandmaster operations leveraging the built-in hardware time stamping on each LAN port of the S650. IEEE 1588 PTP Input LicensePTP input is useful for tunneling time to the S650 over the network. PTP input can be the primary time reference or used as a backup reference in the event of GPS signal loss. With GPS, the S650 can automatically calibrate and store observed network path delay asym-metries for PTP input use if the GPS signal is lost.Multi-GNSS Constellation Support for Enhanced ReliabilityTiming integrity, continuity and reliability can be improved withthe GNSS option that adds support for Galileo, GLONASS, BeiDou, QZSS and SBAS constellations in addition to the standard GPS constellation. With more satellites in view, timing performance can be improved in challenging environments, such as urban canyons. SyncServer S650s ship with GNSS hardware ready to be enabled with a software license. The S650 is also available without GNSS in theS650i model.More Timing I/O StandardThe base S650 can host two modules. The Timing I/O modules are equipped with eight connectors for timing signal input and output. The standard configuration offers a broad yet fixed selection of signal I/Os that include IRIG B, 10 MHz and 1PPS.FlexPort—The Ultimate in Timing FlexibilityOur unique FlexPort technology efficiently and cost-effectively adds innovative “any signal, any connector” capabilities, eliminating the wasted space inherent with legacy style fixed signal modules.The FlexPort option enables the six output connectors (J3-J8) to output many supported signals (time codes, sine waves, program-mable periods) all configurable in real time through the secure web interface. User-entered, nanosecond caliber phase offsets for each connector output accommodates variable cable lengths. The two input connectors (J1-J2) can support a wide variety of input signal types.This level of timing signal flexibility is unprecedented and can even eliminate the need for additional signal distribution chassis as there is no degradation in the precise quality of the coherent signals.Oscillator Upgrades Improve Holdover Accuracy and Save Valuable TimeThe standard S650 is equipped with a crystal oscillator that keeps the S650 accurate to nanoseconds when tracking GPS. However, if GPS connectivity is lost and the server is placed in holdover, the oscillator begins to drift, impacting timing accuracy. Upgrading the oscillator improves the holdover accuracy significantly. For example, consider the following drift rates for the standard oscillator compared to theOCXO and Rubidium upgrades.The value of the upgraded oscillator is that if the GPS signal is lost, the S650 can continue to provide accurate time and frequency. This provides personnel time to correct the problem with only gradualdegradation or disruption in time synchronization accuracy.SpecificationsGNSS Receiver/Antenna• 72 parallel channel GNSS receiver• GPS time traceable to UTC (USNO)• Static and dynamic operational modes• Acquisition time of 30 seconds (cold start)• Cable length up to 900 feet (275 m).• GNSS option adds Galileo/GLONASS/BeiDou/SBAS/QZSS support in addition to GPSTime Accuracy at 1 PPS Output• Standard: <15 ns RMS to UTC (USNO), typical• OCXO: <15 ns RMS to UTC (USNO)• Rubidium: <15 ns RMS to UTC (USNO)After one day locked to GPS; evaluated over normal environment (test range <±5 °F) defined in GR-2830.Oscillator Aging (Monthly)• Standard: ±1×10–7• OCXO: ±5×10–9• Rubidium: ±1×10–10After one month of continuous operation.Holdover Accuracy (One Day)• Standard: 400 µs• OCXO: 25 µs• Rubidium: <1 µsEvaluated over normal environment (test range <±5 °F) defined in GR-2830 after five days locked to GPS.Frequency Output Accuracy and Stability• <1x10–12 at 1 day, after locked to GPS for 1 dayStandard Network Protocols• NTP v3,4 (RFC 1305/5905/8633), SNTP(RFC4330)• NTP v3,4 Symmetric keys: SHA1/256/512 and MD5• SNMP v2c, v3• SNMP MIB II, Custom MIB, system status via SNMP• DHCP/DHCPv6• HTTPS/SSL* (TLS 1.2/1.3)• SMTP forwarding• SSHv2• Telnet• IPv4/IPv6• Syslog: 1 to 8 servers (RFC 3164/5424)• Key management protocols can be individually disabled• Port 1: Management and Time protocols• Port 2, 3 and 4 (optional 5 and 6): Time protocols only Optional Network Protocols NTP Server Performance• 10,000 NTP requests per second while maintaining accuracy associated with reference time source.**• Stratum 1 through GNSS: overall server time stamp accuracy of5 μs to UTC with 1-sigma variation of 20 μs (typical). All NTP timestamps are hardware-based or have real-time hardware compen-sation for internal asymmetric delays. The accuracy is inclusive of all NTP packet delays in and out of the server, as measured at the network interface. NTP serves the UTC timescale by definition,but the user can choose to serve GPS timescale instead. The user can also select the UTC leap second smearing/slewing behavior.The SyncServer easily supports millions of NTP clients.• NTP Activity Charting and MRU Logging: A rolling 24 hour chart displays overall NTPd requests/minute activity. An NTPd MostRecently Used (MRU) list provides details on the most recent 1024 NTP client IP addresses. Data is sortable and exportable. Selec-tion of an individual IP address charts the NTP request totals in 30 minute increments over the past 24 hours. These tools are useful to verify an NTP client is reaching the SyncServer and to identify NTP clients that may be requesting the time more frequently than desired.• NTP Reflector option: 360,000 NTP client mode three requests per second. NTP packets are timestamped 100% in hardware with prevailing clock accuracy. All non-NTP packets are provided to the CPU on a bandwidth-limited basis. The NTP Reflector is included as part of the Security Protocol License option.NTP Activity Chart• Autokey (RFC5906)• PTP• TACACS+• LDAPv3• RADIUS• X.509 certificates for HTTPSand secure syslogRolling 24-hour NTPd activity chart to accompany Most Recently Used (MRU) listwith individual NTPd client activity details and chart.*SSL_High_Encryption Cypher suite or the SSL_High_And_Medium_Encryption Cypher suite.**<5% NTPd packet drop at 10,000 NTPd requests per second. See SyncServer BlueSkyoption data sheet for performance specifications if BlueSky validator is enabled (optional)Mechanical/EnvironmentalShock and VibrationFront PanelRear PanelProduct IncludesS650SyncServer S650 (no option modules installed in base unit), locking power cord, rack mount ears and a two-year hardware warranty. Current manual and MIB are available online at . MIB and REST API can also be downloaded from the SyncServer.S650iSyncServer S650i (no GNSS receiver), one Timing I/O module, locking power cord, rack mount ears and a two-year hardware warranty. Current manual and MIB are available online at . MIB and REST API can also be downloaded from the SyncServer.S650 With Two Standard Timing I/O Modules (Optional Configuration)Ordering InformationCustom configure your build-to-order SyncServer S650 using theonline SyncServer Configurator tool at . Configura -tions can be submitted as requests for quotes.Note: The SyncServer S650 is TAA CompliantNote: The SyncServer S650 is on the DISA/DoDIN Approved Products ListThe Microchip name and logo, the Microchip logo and SyncServer are registered trademarks of Microchip Technology Incorporated in the U.S.A. and other countries. All other trademarks mentioned herein are property of their respective companies. © 2022, Microchip Technology Incorporated and its subsidiaries. All Rights Reserved. 4/22 900-00716-00 Rev N DS00002901FHardware OptionsTiming I/O Module(s)Equipped with eight connectors for timing signal input and output, the standard configuration offers a broad yet fixed selection of signal I/O, including IRIG B, 10 MHz and 1PPS. Five variations of the Timing I/O Module are available as listed below. See the SyncServer Options Datasheet (DS00002920) for more signal types.• Timing I/O Module• Timing I/O Module + Telecom I/O • Timing I/O Module + HaveQuick/PTTI • Timing I/O Module + Fiber Outputs • Timing I/O Module + Fiber Input10 MHz Standard Low Phase Noise ModuleEight isolated, phase-coherent 10 MHz LPN outputs, with excellent levels of LPN and exhibiting low spurious noise characteristics.10 MHz Ultra-Low Phase Noise ModuleSuperior levels of LPN provided on eight extremely isolated, phase-coherent 10 MHz LPN outputs with low spurious noise characteristics.10 GbE LAN PortsTwo additional 10 GbE SFP+ ports equipped with hardware time stamping that supports NTP, PTP and NTP Reflector operations.Rubidium Atomic Oscillator UpgradeImproves stability, accuracy, and holdover accuracy. Holdoveraccuracy is <1 μs for the first 24 hours and <3 μs after the first three days.OCXO Oscillator UpgradeImproves holdover accuracy to 25 μs for the first day.Dual AC Power SuppliesThe dual-corded, dual-AC power supply option provides load sharing and active power management system with hitless failover.Dual DC Power SuppliesThe dual-corded, dual-DC power supply option provides load sharing and active power management system with hitless failover.Antenna AccessoriesAntenna cables and accessories enable versatile solutions to meet most installation requirements.Note: For complete information, see the SyncServer Options Datasheet (DS00002920).Software OptionsSecurity Protocol License with Security-Hardened NTP ReflectorSecurity-hardened NTP Reflector and authentication hardening with NTP Autokey, TACACS+, RADIUS, LDAP and CA-signed X.509 certificates.PTP Output/Grandmaster(Simultaneous Multi-Port/Profile)Single license enables multi-port, multi-profile IEEE 1588 PTP Grand -master operations leveraging the built-in hardware time stamping in all SyncServers.PTP InputPTP as a timing input for tunneling time through PTP or as a backup time reference in the event of the loss of the GNSS signal.FlexPort Technology for Timing I/O ModulesEnables the output connectors to output many supported signals (time codes, sine waves, programmable rates) all configurable in real time. Input connectors can support a wide variety of input signal types.Multi-GNSS ConstellationTrack GPS/SBAS, Galileo, QZSS, GLONASS and/or BeiDou constel-lations for improved integrity and satellite visibility in an urban canyons.1PPS Time Interval/Event Time MeasurementsUse the S650 Timing I/O module to measure, store and statistically display in real time the difference of an external 1PPS relative to the S650. The Event Time capture feature time tags and stores external events.Time-Triggered Programmable PulseProvides a user defined, repetitive and precise time-of-day pulse(s) at specific times or provides periodic, time-based pulse outputs.BlueSky GPS Jamming and Spoofing Detection, Protection, AnalysisDetect GPS jamming and spoofing related anomalies in real-time to protect essential time and frequency outputs.Synchronization SoftwareComprehensive MS Windows-based network time synchronization software with monitoring and auditing functions.Information contained in this publication regarding device applications and the like is provided only for your convenience and may be superseded by updates. It is your responsibility to ensure that your application meets with your specifications. MICROCHIP MAKES NO REPRESENTATIONS OR WARRANTIES OF ANY KIND WHETHER EXPRESS OR IMPLIED, WRITTEN OR ORAL, STATUTORY OR OTHERWISE, RELATED TO THE INFORMATION, INCLUDING BUT NOT LIMITED TO ITS CONDITION, QUALITY, PERFORMANCE, MERCHANTABILITY OR FITNESS FOR PURPOSE. Microchip disclaims all liability arising from this information and its use. Use of Microchip devices in life support and/or safety applications is entirely at the buyer’s risk, and the buyer agrees to defend, indemnify and hold harmless Microchip from any and all damages, claims, suits, or expenses resulting from such use. No licenses are conveyed, implicitly or otherwise, under any Microchip intellectual property rights unless otherwise stated.。
关于拓扑超导的英文演讲

关于拓扑超导的英文演讲Topological superconductivity is a fascinating topic in the field of condensed matter physics that has garnered significant attention in recent years. In this speech, I will provide an overview of the concept, its potential applications, and the ongoing research in this exciting field.Firstly, let's understand what topological superconductivity is. Superconductivity is a quantum phenomenon that occurs at very low temperatures, where certain materials can conduct electricity without any resistance. This property is due to the formation of Cooper pairs, which are pairs of electrons with opposite spins. Topological superconductivity refers to a special class of superconductors where the Cooper pairs exhibit an additional quantum property known as non-Abelian statistics.Non-Abelian statistics means that the quantum wavefunction of the system is not invariant under the exchange of particles. This unique characteristic holds the potential for storing and manipulating quantum information, making topological superconductors a promising platform for developing quantum computers. Unlike conventional superconductors, which are described by Abelian statistics, the non-Abelian nature of topological superconductivity provides protection against certain types of local perturbations and disturbances, making them more stable against noise.The study of topological superconductivity is closely connected to the field of topological insulators. Topological insulators are materials that have a unique electronic band structure that results in conducting surface states while remaining insulating in the bulk. This distinct behavior arises due to the nontrivial topology of the electron wavefunctions. By introducing superconductivity into topological insulators, researchers have been able to realize topological superconductivity.One of the most exciting prospects of topological superconductivity is its potential for hosting Majorana fermions. Majorana fermions are hypothesized particles that are their own antiparticles, meaning they can annihilate and reappear as their own particle. Majorana fermions have distinct properties that make them attractive for quantumcomputing, as they are expected to have a higher resistance to decoherence. Decoherence is a phenomenon that can disrupt quantum states and is a major challenge in quantum computing.Numerous experimental efforts have been dedicated to the search for evidence of Majorana fermions in topological superconductors. One of the most notable experiments is the creation of a hybrid structure called a topological superconductor nanowire. This nanowire, made of materials with strong spin-orbit coupling and proximity-induced superconductivity, exhibits the predicted signatures of Majorana fermions. These experimental advancements have sparked great excitement and sparked further research in the field of topological superconductivity.Apart from quantum computing, topological superconductivity also has potential applications in other areas, such as topological quantum computation and fault-tolerant quantum memories. Researchers are actively exploring the possibilities of using the unique properties of topological superconductors to create new technologies that can revolutionize various fields.In conclusion, topological superconductivity is a captivating area of research with great potential for quantum technologies. Its non-Abelian nature and the possible existence of Majorana fermions make it a promising platform for quantum computing and other applications. Continued experimental efforts and theoretical investigations are crucial in unraveling the mysteries and realizing the full potential of topological superconductivity. The future of this field holds exciting possibilities that could shape the future of quantum technology.。
关于华为突破美国芯片封锁英语作文
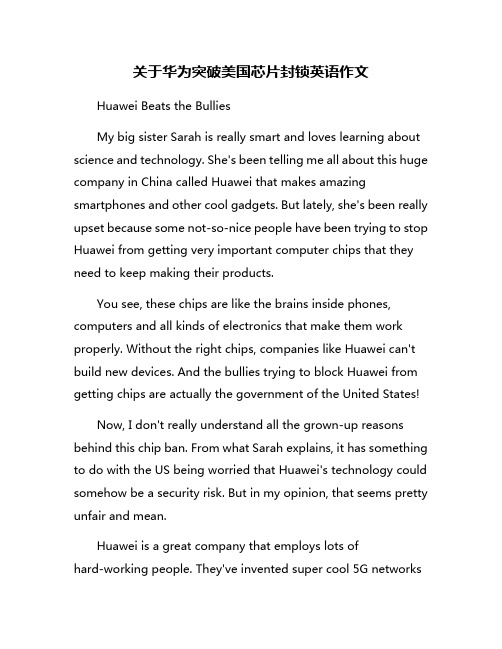
关于华为突破美国芯片封锁英语作文Huawei Beats the BulliesMy big sister Sarah is really smart and loves learning about science and technology. She's been telling me all about this huge company in China called Huawei that makes amazing smartphones and other cool gadgets. But lately, she's been really upset because some not-so-nice people have been trying to stop Huawei from getting very important computer chips that they need to keep making their products.You see, these chips are like the brains inside phones, computers and all kinds of electronics that make them work properly. Without the right chips, companies like Huawei can't build new devices. And the bullies trying to block Huawei from getting chips are actually the government of the United States!Now, I don't really understand all the grown-up reasons behind this chip ban. From what Sarah explains, it has something to do with the US being worried that Huawei's technology could somehow be a security risk. But in my opinion, that seems pretty unfair and mean.Huawei is a great company that employs lots ofhard-working people. They've invented super cool 5G networksthat make our internet blazing fast. Their phones have amazing cameras that can take pictures from far away like bionic eyesight. Huawei even made the first foldable phone that bends in half like a futuristic gadget from the movies! Why would we want to stop such an innovative company from keeping up their great work?Well, those big bullies in the US government don't seem to care about being fair or not hurting innocent people with their actions. By blocking chip suppliers from selling to Huawei,they're trying to cripple the company and make life really difficult. It's so mean and heartless!But you know what? The awesome engineers and scientists at Huawei haven't just given up. They've been working super hard, day and night, to figure out ways to make their own chips so they don't need to rely on anyone else. It's like when you're being picked on by a bully at school - eventually you need to learn to stand up for yourself.From what I've heard from Sarah, Huawei has made incredible strides in developing their own chip technology in a very short period of time. This is despite the bullies constantly trying to throw up new roadblocks and restrictions to slow them down. But the geniuses at Huawei won't be deterred!Just last year, Huawei launched their own Kirin 9000 chipset that is one of the most advanced mobile processors in the world. It's incredibly powerful and energy efficient, letting their new phones have amazing performance and battery life. And get this - Huawei's chips are already being used by other major tech brands like Tesla for their self-driving vehicle systems!More recently, Huawei went even bigger by revealing their brand new Kirin 9000S chipset. This "super" chip takes performance and capabilities to an entirely new level. It's going to be used in Huawei's future flagship phones and devices, letting them stay at the cutting edge of innovation despite the mean bully tactics.Sarah tells me the Kirin 9000S is the first chip to use crazy tiny 3-nanometer transistors, which are so small you'd need a powerful microscope just to see them! That lets Huawei cram way more transistors onto the chip for better computing power than anyone else. The 9000S is also the first mobile chip that can connect to amazingly fast WiFi 7 networks and it has a dedicated computer vision processor for ultra-smart AI cameras.To me, it sounds like a mini super-computer that you can hold in your hand! And Huawei made this incredible technology all by themselves after being so meanly targeted. Instead ofletting the bullies win, they worked harder than ever and came out on top. It just goes to show that brains will always beat brawn, and innovation will prevail over bullying tactics.I'm so proud of the scientists and engineers at Huawei for being brave and persistent in the face of adversity. Thanks to their genius, Huawei can continue thrilling us with cutting-edge technologies for years to come despite those meanies trying to hold them back.In my books, the employees of Huawei are the real champions here. They make me feel hopeful that no matter how mean the bullies get, if youput your mind to it and work really hard, you can overcome any obstacles. Huawei's own struggles prove that world-class innovation will always find a way to break through and keep pushing forward.The bullies might have tried to beat Huawei down, but they just made Huawei even stronger, smarter and more determined in the end. If I learn anything from this story, it's to never give up and never let bullies hold me back from my ambitions. Just study hard, work my brain, and solve problems with creativity and hard work like the heroes at Huawei!。
基于DB-YOLO的双基地雷达弱运动目标检测方法
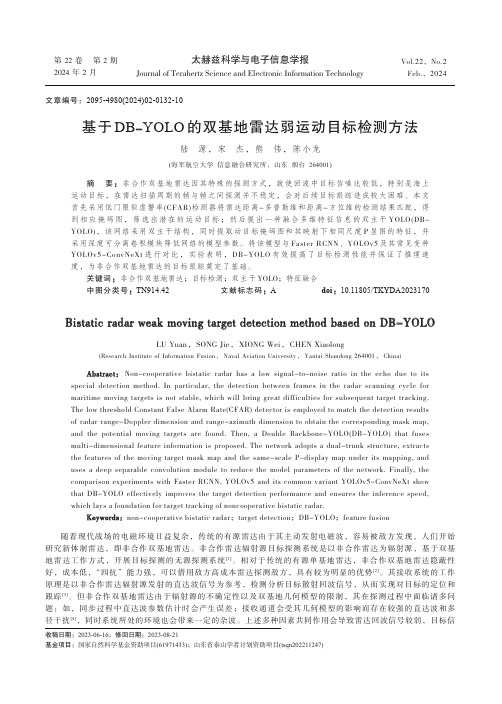
第 22 卷 第 2 期2024 年 2 月太赫兹科学与电子信息学报Journal of Terahertz Science and Electronic Information TechnologyVol.22,No.2Feb.,2024基于DB-YOLO的双基地雷达弱运动目标检测方法陆源,宋杰,熊伟,陈小龙(海军航空大学信息融合研究所,山东烟台264001)摘要:非合作双基地雷达因其特殊的探测方式,致使回波中目标信噪比较低,特别是海上运动目标,在雷达扫描周期的帧与帧之间探测并不稳定,会对后续目标跟踪造成较大困难。
本文首先采用低门限恒虚警率(CFAR)检测器将雷达距离-多普勒维和距离-方位维的检测结果匹配,得到相应掩码图,筛选出潜在的运动目标;然后提出一种融合多维特征信息的双主干YOLO(DB-YOLO),该网络采用双主干结构,同时提取动目标掩码图和其映射下相同尺度P显图的特征,并采用深度可分离卷积模块降低网络的模型参数。
将该模型与Faster RCNN、YOLOv5及其常见变种YOLOv5-ConvNeXt进行对比,实验表明,DB-YOLO有效提高了目标检测性能并保证了推理速度,为非合作双基地雷达的目标跟踪奠定了基础。
关键词:非合作双基地雷达;目标检测;双主干YOLO;特征融合中图分类号:TN914.42 文献标志码:A doi:10.11805/TKYDA2023170Bistatic radar weak moving target detection method based on DB-YOLOLU Yuan,SONG Jie,XIONG Wei,CHEN Xiaolong(Research Institute of Information Fusion,Naval Aviation University,Yantai Shandong 264001,China) AbstractAbstract::Non-cooperative bistatic radar has a low signal-to-noise ratio in the echo due to its special detection method. In particular, the detection between frames in the radar scanning cycle formaritime moving targets is not stable, which will bring great difficulties for subsequent target tracking.The low threshold Constant False Alarm Rate(CFAR) detector is employed to match the detection resultsof radar range-Doppler dimension and range-azimuth dimension to obtain the corresponding mask map,and the potential moving targets are found. Then, a Double Backbone-YOLO(DB-YOLO) that fusesmulti-dimensional feature information is proposed. The network adopts a dual-trunk structure, extractsthe features of the moving target mask map and the same-scale P-display map under its mapping, anduses a deep separable convolution module to reduce the model parameters of the network. Finally, thecomparison experiments with Faster RCNN, YOLOv5 and its common variant YOLOv5-ConvNeXt showthat DB-YOLO effectively improves the target detection performance and ensures the inference speed,which lays a foundation for target tracking of noncooperative bistatic radar.KeywordsKeywords::non-cooperative bistatic radar;target detection;DB-YOLO;feature fusion 随着现代战场的电磁环境日益复杂,传统的有源雷达由于其主动发射电磁波,容易被敌方发现,人们开始研究新体制雷达,即非合作双基地雷达。
量子仿真综述

arXiv:1402.1388v2 [cond-mat.mes-heived: date / Accepted: date
Abstract Quantum systems are notoriously difficult to simulate with classical means. Recently, the idea of using another quantum system - which is experimentally more controllable - as a simulator for the original problem has gained significant momentum. Amongst the experimental platforms studied as quantum simulators, superconducting qubits are one of the most promising, due to relative straightforward scalability, easy design, and integration with standard electronics. Here I review the recent state-of-the art in the field and the prospects for simulating systems ranging from relativistic quantum fields to quantum many-body systems. Keywords Josephson devices · digital and analog quantum simulation · many-body systems · quantum fields · circuit QED 1 Introduction The idea of extracting information about one quantum system by mathematically mapping it to another one which is more easily accessible goes back to Richard Feynman [1] and David Deutsch [2]. The development of the field of quantum simulation is intertwined with that of quantum computing, since from the beginning quantum simulation was envisioned as the main application of a quantum computer. A universal quantum computer would be able to efficiently simulate the dynamics of quantum systems, provided that the Hamiltonian is built up from local interactions [3]. Powerful mathematical results such as the
白皮书:PERC Point-to-Point Resistance(P2P resistance)
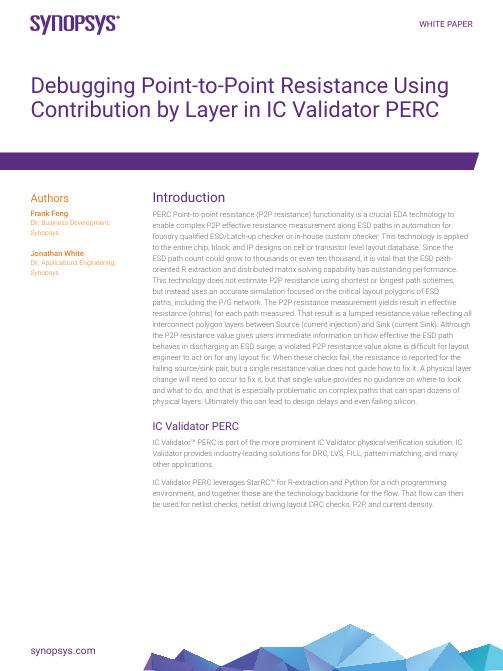
WHITE PAPER Introduction PERC Point-to-point resistance (P2P resistance) functionality is a crucial EDA technology to enable complex P2P effective resistance measurement along ESD paths in automation forfoundry qualified ESD/Latch-up checker or in-house custom checker. This technology is appliedto the entire chip, block, and IP designs on cell or transistor level layout database. Since theESD path count could grow to thousands or even ten thousand, it is vital that the ESD path-oriented R extraction and distributed matrix solving capability has outstanding performance.This technology does not estimate P2P resistance using shortest or longest path schemes,but instead uses an accurate simulation focused on the critical layout polygons of ESDpaths, including the P/G network. The P2P resistance measurement yields result in effectiveresistance (ohms) for each path measured. That result is a lumped resistance value reflecting allinterconnect polygon layers between Source (current injection) and Sink (current Sink). Althoughthe P2P resistance value gives users immediate information on how effective the ESD pathbehaves in discharging an ESD surge, a violated P2P resistance value alone is difficult for layoutengineer to act on for any layout fix. When these checks fail, the resistance is reported for thefailing source/sink pair, but a single resistance value does not guide how to fix it. A physical layerchange will need to occur to fix it, but that single value provides no guidance on where to lookand what to do, and that is especially problematic on complex paths that can span dozens ofphysical layers. Ultimately this can lead to design delays and even failing silicon. IC Validator PERCIC Validator™ PERC is part of the more prominent IC Validator physical verification solution. ICValidator provides industry-leading solutions for DRC, LVS, FILL, pattern matching, and manyother applications.IC Validator PERC leverages StarRC™ for R-extraction and Python for a rich programmingenvironment, and together those are the technology backbone for the flow. That flow can thenbe used for netlist checks, netlist driving layout DRC checks, P2P , and current density.AuthorsFrank FengDir, Business Development,Synopsys Jonathan White Dir, Applications Engineering, SynopsysDebugging Point-to-Point Resistance Using Contribution by Layer in IC Validator PERCIC ValidatorFigure 1: IC Validator Physical Verification SolutionIC Validator PERC is qualified for significant foundry ESD/LUP checking of P2P resistance measurement, even at the full-chip level. IC Validator PERC P2P flow employs StarRC for R extraction, which is the industry gold standard. To provide better P2P resistance analysis for the layout engineer to act when there is a P2P resistance violation, IC Validator PERC offers a distinctive featureto analyze P2P resistance result contribution by layer. This feature enables beneficial information for the user to decide which interconnect layers are high contributors to be the candidates for layout fix.Enabling IC Validator PERC Collecting Database for P2P Resistance Contribution by Layer To use this debug capability, R reduction will need to be turned off in the P2P resistance flow. The reason is that reduction greatly simplifies the R network, and information about the actual fractured layout polygons is lost. This control is in the StarRC tech file, and the IC Validator PERC flow has a mechanism to define rules to PERC that then get passed into StarRC. The “readme” for your foundry runset for P2P resistance would provide the information needed to do this.Performance Impact of Producing Data for P2P Resistance Contribution by LayerIf the user is only running PERC P2P on IO nets and other non-P/G nets, then enabling P2P resistance contribution by layer will have minimal impact on performance. However, if the user is running PERC P2P, including P/G nets, then the user should expecta performance impact, depending on the size of the design. This performance impact is insignificant if the design is small (chip size is less than a few mm^2). If the chip size is larger than 10 mm^2, the performance cost will be more significant due to StarRC not reducing the R network of the P/G nets. The chip size estimation for performance cost is a rough guide to keep in mind andnot a hard rule.Using IC Validator VUE to Access P2P Resistance Contribution by Layer in Conjunction with Layout HighlightingThe user can launch IC Validator PERC job as usual. Upon the successful completion of IC Validator PERC P2P job, the user will be able to analyze P2P resistance contribution by layer in IC Validator VUE together with a layout viewer supported by IC Validator VUE. In the below section, the debug process in conjunction with P2P resistance contribution by layer is described. The user starts a layout viewer such as IC Validator WorkBench, Virtuoso, or Custom Compiler™. With the layout database open, the user invokes IC Validator VUE and loads topcell.vue file. Select “PERC Errors” tab to point to IC Validator PERC P2P run results; the violation P2P paths associated with each check name are listed on the Violation Browser page (on the left panel of VUE main window). Select one of the paths, and more details are shown as Violation Details/Description (on the right panel of VUE main window). Select the top line for each path in the Violation Details panel, right-click to drop-down list of can-do function, select (left click) on “PERC Path Heatmap,” and then a “Highlight Path” dialog window pops out. In the top portion of “Highlight Path” dialog window, there are list of symbols. Select the rightmost symbol (looks like a table), the P2P resistance contribution by layer table named “Contributionto Path Resistance” is shown. Each of these steps in the VUE debug procedures to access P2P resistance contribution by layer is displayed in figure 2.Figure 2: A flow chart describes accessing P2P resistance contribution by layer in VUE.The P2P resistance contribution by layer data (named as Contribution to Path Resistance table) provides how the lumped total resistance of the selected P2P path is summed up from various layers. Since each layer has its sheet resistance, layout polygons alone can’t tell the user what to do. The top contributors of resistance combined with layer polygon highlight capability in the “Highlight Path” dialog window give the layout engineer a much better idea of what to do for a layout fix. Figure 3 shows one P2P path measured from ESD diodes one physical power Pad of a power net.©2021 Synopsys, Inc. All rights reserved. Synopsys is a trademark of Synopsys, Inc. in the United States and other countries. A list of Synopsys trademarks is availableat /copyright .html . All other names mentioned herein are trademarks or registered trademarks of their respective owners.Figure 3: Pictures show how P2P resistance contribution by layer table provides valuable information for a user to focus debug and layout fix priorityInterpreting Contribution by Layer Results to Fix Design IssuesFixing P2P resistance issues in a design can be a complex problem. Proper fixing depends most heavily on the designer’s knowledge of their design and what changes they can make to resolve it. Additional information like a contribution by layer is intended to help understand the results more effectively so that the designer can apply their design knowledge with more confidence and greater speed. So, for example, knowing that the top thick metal represents 70% of the P2P resistance contribution does show what to change with that metal routing. But it does indicate quickly to a design whether the results are as expected or whether something unusual has occurred. And it gives confidence for the designer to make changes to the top think metal knowing that there will not be unintended consequences for that changes.SummaryPoint-to-point resistance checking is an essential component of robust ESD design verification. However, debugging the reported errors can be a real challenge and frustration to ESD engineers. IC Validator PERC provides the “contribution by layer” feature in its P2P Heatmap interface to IC Validator VUE, which offers tremendous insight into fixing these critical design errors. This saves time in the design cycle and gives higher confidence going into silicon ESD testing.。
Study on cooling process of cryogenic system for superconducting magnets of BEPCⅡ
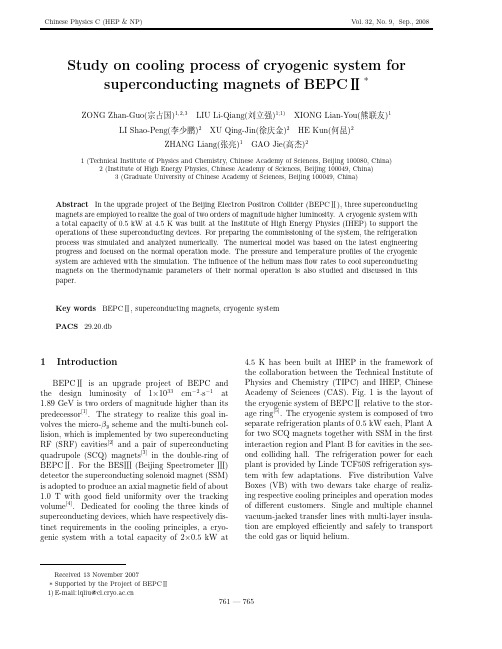
Chinese Physics C(HEP&NP)Vol.32,No.9,Sep.,2008Study on cooling process of cryogenic system for superconducting magnets of BEPC *ZONG Zhan-Guo( )1,2,3LIU Li-Qiang( )1;1)XIONG Lian-You( )1LI Shao-Peng( )2XU Qing-Jin( )2HE Kun( )2ZHANG Liang( )1GAO Jie( )21(Technical Institute of Physics and Chemistry,Chinese Academy of Sciences,Beijing100080,China) 2(Institute of High Energy Physics,Chinese Academy of Sciences,Beijing100049,China)3(Graduate University of Chinese Academy of Sciences,Beijing100049,China) Abstract In the upgrade project of the Beijing Electron Positron Collider(BEPC ),three superconducting magnets are employed to realize the goal of two orders of magnitude higher luminosity.A cryogenic system witha total capacity of0.5kW at4.5K was built at the Institute of High Energy Physics(IHEP)to support theoperations of these superconducting devices.For preparing the commissioning of the system,the refrigeration process was simulated and analyzed numerically.The numerical model was based on the latest engineering progress and focused on the normal operation mode.The pressure and temperature profiles of the cryogenic system are achieved with the simulation.The influence of the helium massflow rates to cool superconducting magnets on the thermodynamic parameters of their normal operation is also studied and discussed in this paper.Key words BEPC ,superconducting magnets,cryogenic systemPACS29.20.db1IntroductionBEPC is an upgrade project of BEPC and the design luminosity of1×1033cm−2·s−1at 1.89GeV is two orders of magnitude higher than its predecessor[1].The strategy to realize this goal in-volves the micro-βy scheme and the multi-bunch col-lision,which is implemented by two superconducting RF(SRF)cavities[2]and a pair of superconducting quadrupole(SCQ)magnets[3]in the double-ring of BEPC .For the BES (Beijing Spectrometer ) detector the superconducting solenoid magnet(SSM) is adopted to produce an axial magneticfield of about 1.0T with goodfield uniformity over the tracking volume[4].Dedicated for cooling the three kinds of superconducting devices,which have respectively dis-tinct requirements in the cooling principles,a cryo-genic system with a total capacity of2×0.5kW at 4.5K has been built at IHEP in the framework of the collaboration between the Technical Institute of Physics and Chemistry(TIPC)and IHEP,Chinese Academy of Sciences(CAS).Fig.1is the layout of the cryogenic system of BEPC relative to the stor-age ring[5].The cryogenic system is composed of two separate refrigeration plants of0.5kW each,Plant A for two SCQ magnets together with SSM in thefirst interaction region and Plant B for cavities in the sec-ond colliding hall.The refrigeration power for each plant is provided by Linde TCF50S refrigeration sys-tem with few adaptations.Five distribution Valve Boxes(VB)with two dewars take charge of realiz-ing respective cooling principles and operation modes of different customers.Single and multiple channel vacuum-jacked transfer lines with multi-layer insula-tion are employed efficiently and safely to transport the cold gas or liquid helium.762Chinese Physics C(HEP&NP)Vol.32No.9ZONG Zhan-Guo et al Study on cooling process of cryogenic system for superconducting magnets of BEPC 763items heat loads unit Single-phase(the vapor quality:x<0.01or x>0.9)pressure drops along the cryogenic transfer lines is calculated according to the Darcy friction fac-tor.This factor(as represented on a Moody chart[10]) is as a function of Reynolds numbers(Re)for laminar flow,and as a function of both Reynolds number and wall roughness ratio(roughness over diameter,e/D) for turbulentflow.The pressure drops in two phase(i. e.,vapor-liquid)flow can be dramatically higher than the pure liquidflow at the same overall massflux,easily one or two orders of magnitude higher.Two-phase multi-pliers have been used to account for this and provide a simple means of estimating the relative increase in pressure drop due to the presence of the vapor phase. In this study the two-phase multipliers for the two phase heliumflows are predicted by the simplest ho-mogeneous model,where both phases are assumed to flow with the same average velocity[11].The equations proposed by McAdams et al.[12]in1942are used to evaluate the mixture dynamic viscosity(µ)and den-sity(ρ)of two-phase heliumflow.For the full open ball valves,the equivalent length is340times of their diameters[13].Pressure drops in the cooling channels of superconducting magnets are neglected.For the performance of heat exchangers in the1000L and60L dewars,the calculation is con-ducted by Ref.[14].The refrigerator is assumed to provide the helium of setting conditions in the sta-ble and automatic operation.The thermo physical data of helium are taken from the standard helium property code HEPAK[15].The quasi-Newton iterative and downhill methods[16]are adopted in the computing process in which the massflow rates and the pressure or pressure drop over the control valves are chosen as the independent variables to construct the functions in accordance with the mass balances of the liquid containers and the equal pressures at the point of pipes’inter-junctions.4Simulation results and discussion Figure3provides the pressure(a)and tempera-ture(b)profiles of the cryogenic system for the mag-nets.The total massflow rate for three magnets is 31.58g/s and is sub-cooled to4.507K at0.262MPa by the heat exchanger in the1000L dewar.Under theflow rate of11.997g/s for the SCQ-A and13.8g/s for the SCQ-B,they can both operate at the average temperature of4.75K and the temperature rise of heliumflow through magnets is around0.2K.The pressures and temperatures of two branches for the SCQ magnets have slight difference due to different lengths of the cryogenic transfer lines but both can meet the normal operation requirements.Just out of the SCQ magnets,the helium is further throttled to release the pressure by a control valve.As the SSM is situated far away from the refrig-erator,theflow rate of6.1g/s has a temperature rise along its long cryogenic transfer lines.The pre-cooler in the60L dewar is effective to eliminate the rise764Chinese Physics C(HEP&NP)Vol.32No.9ZONG Zhan-Guo et al Study on cooling process of cryogenic system for superconducting magnets of BEPC 765References1BEPC Group.Design Report of BEPC .Beijing:Insti-tute of High Energy Physics,Chinese Academy of Sciences, 20022LI Z Q.Study on Optimization Design of Superconduct-ing RF Cavities for BEPC .Ph.D.Dissertation.Beijing: Institute of High Energy Physics,Chinese Academy of Sci-ences,2003(in Chinese)3CHEN F S.Design of Special Type Magnet and Study of Quench Protection System for BEPC Interaction Re-gion.Ph.D.Dissertation.Beijing:Institute of High Energy Physics,Chinese Academy of Sciences,2006(in Chinese) 4ZHU Z A,ZHAO L et al.The BES Detector Magnet.Proceedings of ICEC20.Oxford:Eisevier,2005.593—596 5BEPC Group.IHEP-BEPC -SB-03-5.Beijing:Institute of High Energy Physics,Chinese Academy of Sciences,Nov.20036BNL Magnet Division.Design Report for the BEPC Su-perconducting IR Magnets,AM-NO-328.Brookhaven Na-tional Laboratory,20037Lierl H.Technology of Cryogenic for Storage Rings.Pro-ceedings of EPAC98,Stockholm.Sweden,1998.194—1998XU Q J,ZHU Z A et al.Thermal Design of BES Magnet.Proceedings of ICEC20,Oxford:Eisevier,2005.601—603 9XU Q J.Thermal Analysis for the Detector Superconduct-ing Magnets in High Energy Physics Field.Ph.D.Disserta-tion.Beijing:Technical Institute of Physics and Chemistry, Chinese Academy of Sciences,2006(in Chinese)10White Frank M.Fluid Mechanics.McGraw-Hill,1979.332—34111Van Sciver W.Helium Cryogenics.New York:Plenum Press,1986.256—26012McAdams W H,Woods W K,Bryan R L.Vaporization Inside Horizontal Tubes II.Benzene-oil Mixtures,Trans.1942,ASME64.19313Weisend II J G.Handbook of Cryogenic Engineering.Tay-lor&Francis,1998.370—37114YANG S M,TAO W Q.Heat Transfer.Beijing:Higher Education Press,1998.332—335(in Chinese)15HEPAK(©copyright Cryodata Inc.)is a Computer Pro-gram for Calculating the Thermo Physical Properties of Helium.Horizon Technology,version3.4,Colorado,USA 16DENG J Z et puting Method.Xi’an:Xi’an Jiaotong University Press,1985(in Chinese)。
贝叶斯超参数优化 多层感知器
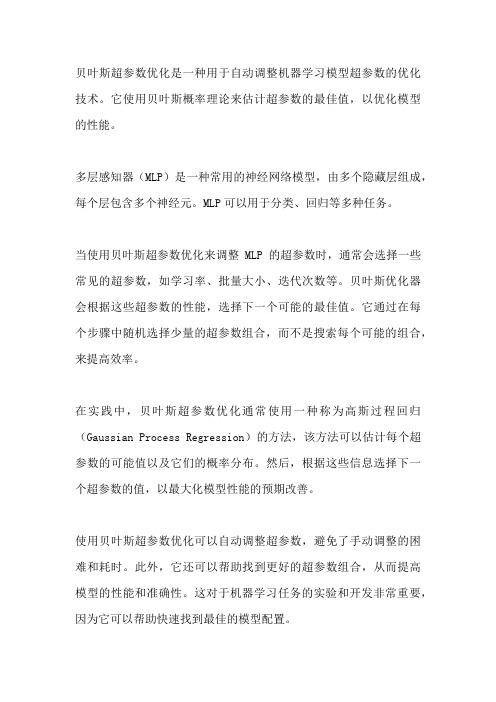
贝叶斯超参数优化是一种用于自动调整机器学习模型超参数的优化技术。
它使用贝叶斯概率理论来估计超参数的最佳值,以优化模型的性能。
多层感知器(MLP)是一种常用的神经网络模型,由多个隐藏层组成,每个层包含多个神经元。
MLP可以用于分类、回归等多种任务。
当使用贝叶斯超参数优化来调整MLP的超参数时,通常会选择一些常见的超参数,如学习率、批量大小、迭代次数等。
贝叶斯优化器会根据这些超参数的性能,选择下一个可能的最佳值。
它通过在每个步骤中随机选择少量的超参数组合,而不是搜索每个可能的组合,来提高效率。
在实践中,贝叶斯超参数优化通常使用一种称为高斯过程回归(Gaussian Process Regression)的方法,该方法可以估计每个超参数的可能值以及它们的概率分布。
然后,根据这些信息选择下一个超参数的值,以最大化模型性能的预期改善。
使用贝叶斯超参数优化可以自动调整超参数,避免了手动调整的困难和耗时。
此外,它还可以帮助找到更好的超参数组合,从而提高模型的性能和准确性。
这对于机器学习任务的实验和开发非常重要,因为它可以帮助快速找到最佳的模型配置。
金的等离子共振等综述
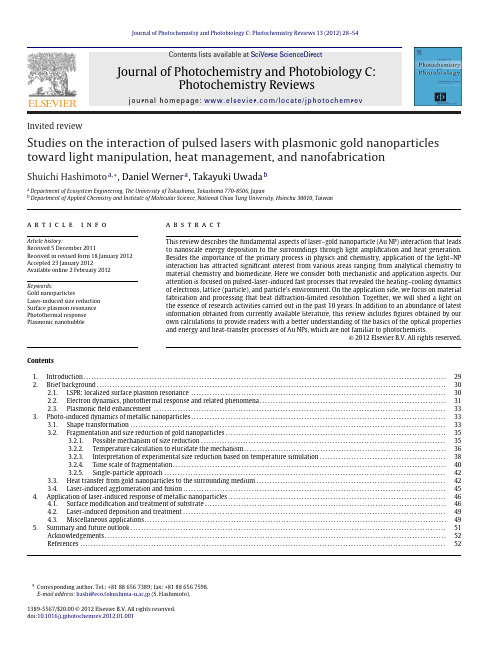
a b s t r a c t
This review describes the fundamental aspects of laser–gold nanoparticle (Au NP) interaction that leads to nanoscale energy deposition to the surroundings through light amplification and heat generation. Besides the importance of the primary process in physics and chemistry, application of the light–NP interaction has attracted significant interest from various areas ranging from analytical chemistry to material chemistry and biomedicine. Here we consider both mechanistic and application aspects. Our attention is focused on pulsed-laser-induced fast processes that revealed the heating–cooling dynamics of electrons, lattice (particle), and particle’s environment. On the application side, we focus on material fabrication and processing that beat diffraction-limited resolution. Together, we will shed a light on the essence of research activities carried out in the past 10 years. In addition to an abundance of latest information obtained from currently available literature, this review includes figures obtained by our own calculations to provide readers with a better understanding of the basics of the optical properties and energy and heat-transfer processes of Au NPs, which are not familiar to photochemists. © 2012 Elsevier B.V. All rights reserved.
CURRENT LEAD FOR SUPERCONDUCTING EQUIPMENT
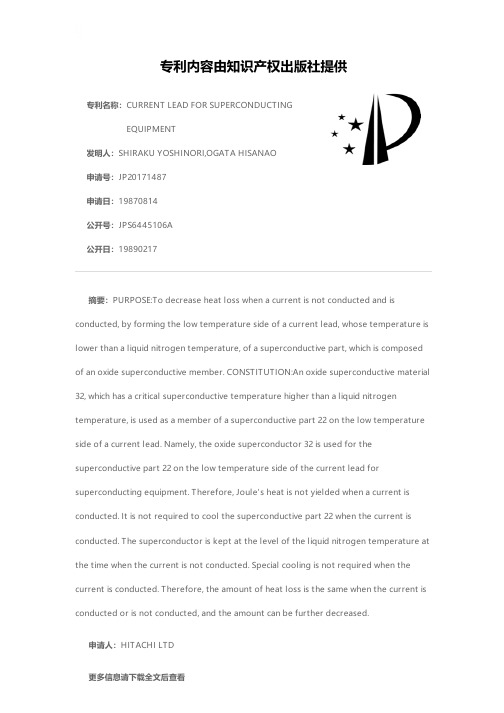
专利名称:CURRENT LEAD FOR SUPERCONDUCTING PMENT
发明人:SHIRAKU YOSHINORI,OGATA HISANAO 申请号:J P 201714 87 申请日:19870814 公开号:J P S64 4 5106A 公开日:19890217
申请人:HITACHI LTD
更多信息请下载全文后查看
摘要:PURPOSE:To decrease heat loss when a current is not conducted and is conducted, by forming the low temperature side of a current lead, whose temperature is lower than a liquid nitrogen temperature, of a superconductive part, which is composed of an oxide superconductive member. CONSTITUTION:An oxide superconductive material 32, which has a critical superconductive temperature higher than a liquid nitrogen temperature, is used as a member of a superconductive part 22 on the low temperature side of a current lead. Namely, the oxide superconductor 32 is used for the superconductive part 22 on the low temperature side of the current lead for superconducting equipment. Therefore, Joule's heat is not yielded when a current is conducted. It is not required to cool the superconductive part 22 when the current is conducted. The superconductor is kept at the level of the liquid nitrogen temperature at the time when the current is not conducted. Special cooling is not required when the current is conducted. Therefore, the amount of heat loss is the same when the current is conducted or is not conducted, and the amount can be further decreased.
super barrier rectifier介绍 -回复
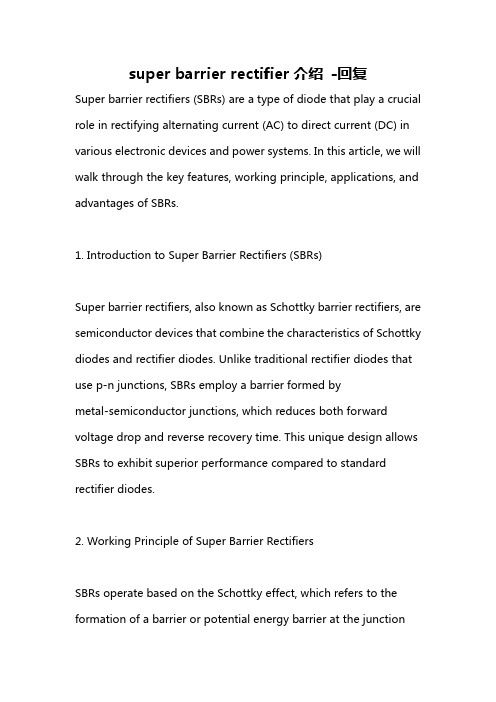
super barrier rectifier介绍-回复Super barrier rectifiers (SBRs) are a type of diode that play a crucial role in rectifying alternating current (AC) to direct current (DC) in various electronic devices and power systems. In this article, we will walk through the key features, working principle, applications, and advantages of SBRs.1. Introduction to Super Barrier Rectifiers (SBRs)Super barrier rectifiers, also known as Schottky barrier rectifiers, are semiconductor devices that combine the characteristics of Schottky diodes and rectifier diodes. Unlike traditional rectifier diodes that use p-n junctions, SBRs employ a barrier formed bymetal-semiconductor junctions, which reduces both forward voltage drop and reverse recovery time. This unique design allows SBRs to exhibit superior performance compared to standard rectifier diodes.2. Working Principle of Super Barrier RectifiersSBRs operate based on the Schottky effect, which refers to the formation of a barrier or potential energy barrier at the junctionbetween a metal and a semiconductor material. When aforward-bias voltage is applied to the SBR, current flows easily through the metal-semiconductor junction due to the reduced resistance, resulting in low forward voltage drop. On the other hand, when a reverse-bias voltage is applied, the barrier prevents the flow of current from the metal to the semiconductor, leading to a high reverse breakdown voltage and minimal leakage current.3. Key Features and Advantages of Super Barrier RectifiersSuper barrier rectifiers offer several advantages over traditional rectifier diodes, making them highly preferred in various applications. Some of the key features and advantages include:3.1 Low Forward Voltage Drop: SBRs typically have a lower forward voltage drop compared to standard rectifier diodes. This attribute helps reduce power losses and improves overall system efficiency.3.2 Fast Switching Speed: Due to the absence of minority carrier injection and storage time, SBRs exhibit faster switching speeds and significantly reduced reverse recovery time, leading to improved system performance.3.3 High Temperature Operation: SBRs are designed to operate over a wide temperature range, making them suitable for use in high-temperature environments.3.4 Low Power Loss: The reduced forward voltage drop of SBRs contributes to lower power loss, enabling energy-efficient systems.4. Applications of Super Barrier RectifiersSuper barrier rectifiers find applications in a wide range of electronic devices and power systems. Some of the common applications include:4.1 Power Supplies: SBRs are widely used in power supply circuits, especially in applications that require high-efficiency conversion from AC to DC, such as adapters, chargers, and industrial power equipment.4.2 Automotive Electronics: Due to their fast switching speed and low power loss, SBRs are suitable for use in automotive electronics, including LED lighting, automotive control circuits, and batterycharging systems.4.3 Solar Power Systems: SBRs are employed in solar power systems to convert the generated AC power into DC power for use in residential, commercial, and industrial applications.4.4 Consumer Electronics: SBRs are utilized in various consumer electronic devices, such as computers, mobile phones, televisions, and audio equipment, to rectify power supply and enable efficient operation.5. ConclusionSuper barrier rectifiers (SBRs) are innovative semiconductor devices that offer several advantages over traditional rectifier diodes. Their low forward voltage drop, fast switching speed, high-temperature operation, and low power loss make them exceptionally suitable for a wide range of applications, including power supplies, automotive electronics, solar power systems, and consumer electronics. As technology continues to evolve, SBRs are expectedto play an increasingly significant role in improving energy efficiency and performance in electrical and electronic systems.。
突触中信号转换的方式
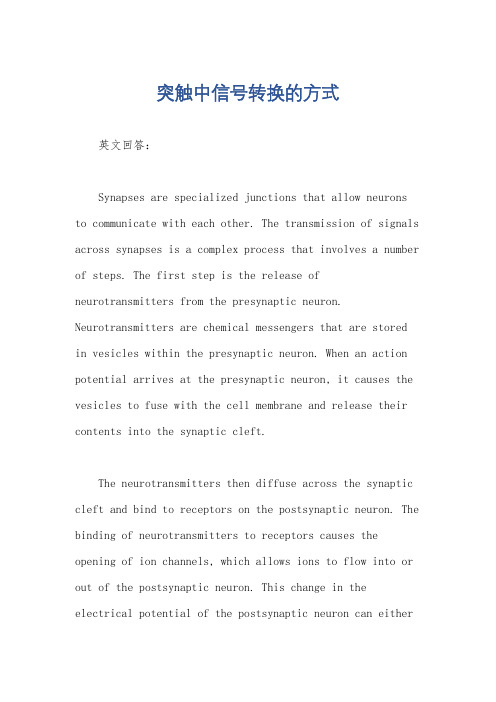
突触中信号转换的方式英文回答:Synapses are specialized junctions that allow neurons to communicate with each other. The transmission of signals across synapses is a complex process that involves a number of steps. The first step is the release of neurotransmitters from the presynaptic neuron. Neurotransmitters are chemical messengers that are stored in vesicles within the presynaptic neuron. When an action potential arrives at the presynaptic neuron, it causes the vesicles to fuse with the cell membrane and release their contents into the synaptic cleft.The neurotransmitters then diffuse across the synaptic cleft and bind to receptors on the postsynaptic neuron. The binding of neurotransmitters to receptors causes the opening of ion channels, which allows ions to flow into or out of the postsynaptic neuron. This change in theelectrical potential of the postsynaptic neuron can eitherexcite or inhibit the neuron, causing it to produce an action potential or not, respectively.The transmission of signals across synapses is a highly regulated process. The amount of neurotransmitter that is released, the affinity of the neurotransmitter for its receptor, and the number of receptors on the postsynaptic neuron are all factors that can affect the strength of the synaptic signal. In addition, synapses can be modified over time through a process known as synaptic plasticity. Synaptic plasticity allows the strength of synaptic connections to be strengthened or weakened, which is essential for learning and memory.中文回答:突触是神经元之间进行交流的特殊连接点。
pinn反演激光烧蚀参数
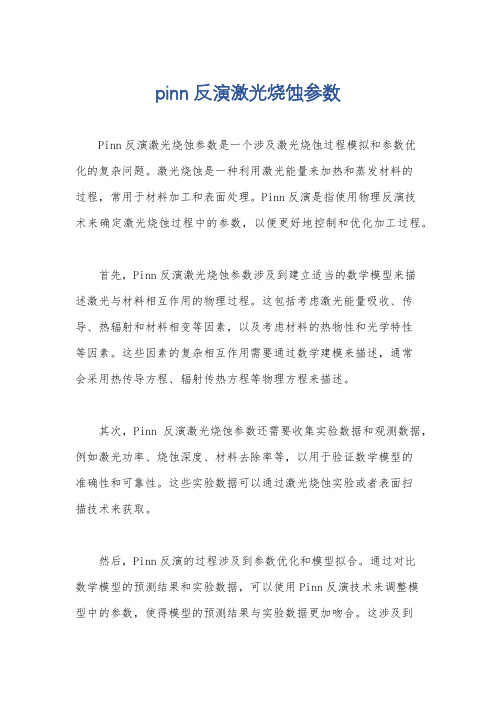
pinn反演激光烧蚀参数Pinn反演激光烧蚀参数是一个涉及激光烧蚀过程模拟和参数优化的复杂问题。
激光烧蚀是一种利用激光能量来加热和蒸发材料的过程,常用于材料加工和表面处理。
Pinn反演是指使用物理反演技术来确定激光烧蚀过程中的参数,以便更好地控制和优化加工过程。
首先,Pinn反演激光烧蚀参数涉及到建立适当的数学模型来描述激光与材料相互作用的物理过程。
这包括考虑激光能量吸收、传导、热辐射和材料相变等因素,以及考虑材料的热物性和光学特性等因素。
这些因素的复杂相互作用需要通过数学建模来描述,通常会采用热传导方程、辐射传热方程等物理方程来描述。
其次,Pinn反演激光烧蚀参数还需要收集实验数据和观测数据,例如激光功率、烧蚀深度、材料去除率等,以用于验证数学模型的准确性和可靠性。
这些实验数据可以通过激光烧蚀实验或者表面扫描技术来获取。
然后,Pinn反演的过程涉及到参数优化和模型拟合。
通过对比数学模型的预测结果和实验数据,可以使用Pinn反演技术来调整模型中的参数,使得模型的预测结果与实验数据更加吻合。
这涉及到优化算法和数值计算方法的应用,以找到最优的参数组合。
最后,Pinn反演激光烧蚀参数的应用包括优化激光加工过程的参数设定,提高加工质量和效率,以及探索新的材料和激光加工工艺。
这对于制造业和材料加工领域具有重要意义。
总之,Pinn反演激光烧蚀参数涉及到物理建模、实验数据采集、参数优化和应用等多个方面,是一个复杂而重要的研究课题。
通过Pinn反演技术,可以更好地理解和控制激光烧蚀过程,推动激光加工技术的发展和应用。
- 1、下载文档前请自行甄别文档内容的完整性,平台不提供额外的编辑、内容补充、找答案等附加服务。
- 2、"仅部分预览"的文档,不可在线预览部分如存在完整性等问题,可反馈申请退款(可完整预览的文档不适用该条件!)。
- 3、如文档侵犯您的权益,请联系客服反馈,我们会尽快为您处理(人工客服工作时间:9:00-18:30)。
a r X i v :c o n d -m a t /0303569v 1 [c o n d -m a t .s u p r -c o n ] 27 M a r 2003Sb-NQR probe for superconducting property in the Pr-based filled skutteruditecompound PrRu 4Sb 12M.Yogi 1,H.Kotegawa 1,Y.Imamura 1,G.-q.Zheng 1,Y.Kitaoka 1,H.Sugawara 2,H.Sato 21Department of Physical Science,Graduate School of Engineering Science,Osaka University,Osaka 560-8531,Japan and2Graduate School of Science,Tokyo Metropolitan University,Minami-Ohsawa 1-1,Hachioji,Tokyo 192-0397,Japan(Dated:February 2,2008)We report the electronic and superconducting properties in the Pr-based filled-skutterudite su-perconductor PrRu 4Sb 12with T c =1.3K via the measurements of nuclear-quadrupole-resonance (NQR)frequency νQ and nuclear-spin-lattice-relaxation time T 1of Sb nuclei.The temperature de-pendence of νQ has revealed the energy scheme of Pr 3+crystal electric field (CEF)that is consistent with an energy separation ∆CEF ∼70K between the ground state and the first-excited state.In the normal state,the Korringa relation of (1/T 1T )P r =const.is valid,with [(1/T 1T )P r /(1/T 1T )La ]1/2∼1.44where (1/T 1T )La is for LaRu 4Sb 12.These results are understood in terms of a conventional Fermi liquid picture in which the Pr-4f 2state derives neither magnetic nor quadrupolar degrees of freedom at low temperatures.In the superconducting state,1/T 1shows a distinct coherence peak just below T c ,followed by an exponential decrease with a value of 2∆/k B T c =3.1.These results demonstrate that PrRu 4Sb 12is a typical weak-coupling s -wave superconductor,in strong contrast with the heavy-fermion superconductor PrOs 4Sb 12that is in an unconventional strong coupling regime.The present study on PrRu 4Sb 12highlights that the Pr-4f 2derived non-magnetic doublet plays a key role in the unconventional electronic and superconducting properties in PrOs 4Sb 12.PACS numbers:71.27.+a,76.60.-kFilled-skutterudite compounds ReT 4Pn 12(Re =rare earth;T =Fe,Ru and Os;Pn =pnictogen)show rich properties.PrRu 4P 12and PrFe 4P 12show a metal-insulator transition and undergo into an anoma-lous heavy-fermion (HF)state,respectively,whereas PrRu 4As 12,PrRu 4Sb 12and PrOs 4Sb 12exhibit a super-conducting (SC)transition.1,2,3,4Bauer et al.reported that PrOs 4Sb 12shows HF behavior and superconducts at T c =1.85K.It is the first Pr-based HF superconductor.4Its HF state was inferred from the jump in the spe-cific heat at T c ,the slope of the upper critical field H c 2near T c ,and the electronic specific-heat coefficient γ∼350−500mJ/mole K 2.Magnetic susceptibil-ity,thermodynamic measurements,and inelastic neutron scattering experiments revealed the ground state of the Pr 3+ions in the cubic crystal electric field (CEF)to be the Γ3nonmagnetic doublet 4,5.In the Pr-based com-pounds with the Γ3ground state,electric quadrupolar interactions play an important role.In analogy with a quadrupolar Kondo model,6it was suggested that the HF-like behavior exhibited by PrOs 4Sb 12may have some-thing to do with a Pr-4f 2-derived quadrupolar Kondo-lattice.An interesting issue to be addressed is what role of Pr-4f 2-derived quadrupolar fluctuations plays in rel-evance with the onset of the superconductivity in this compound.Meanwhile,Kotegawa et al.have reported the Sb-NQR results which evidence the HF behavior and the unconventional SC property in PrOs 4Sb 12.7The temper-ature (T )dependencies of nuclear-spin-lattice-relaxation rate,1/T 1and nuclear-quadrupole-resonance (NQR)fre-quency unraveled a low-lying CEF splitting below T 0∼10K,associated with the Pr 3+(4f 2)-derived ground state.The analysis of T 1suggests the formation of HF state below ∼4K.In the SC state,1/T 1shows neither a coherence peak just below T c =1.85K nor a T 3like power-law behavior observed for anisotropic HF super-conductors with line-node gap.An isotropic energy-gap with ∆/k B =4.8K is suggested to open up already be-low T ∗∼2.3K.It is surprising that PrOs 4Sb 12looks like an isotropic HF superconductor –it may indeed argue for Cooper pairing via quadrupolar fluctuations.Also,PrRu 4Sb 12was reported to undergo a SC transition at T c =1.3K from the measurements of the electrical re-sistivity and specific heat as well as LaRu 4Sb 12with T c =3.58K.3It can be informative to compare PrRu 4Sb 12with PrOs 4Sb 12and the related La-based superconduc-tors as shown in Table I.8The localized character of 4f electrons,namely the closeness of the respective Fermi surfaces with those in LaRu 4Sb 12and LaOs 4Sb 12,has been confirmed in PrRu 4Sb 12and PrOs 4Sb 12based on the de Haas-van Alphen (dHvA)experiment.8,13On the contrary,the mass enhancement in PrRu 4Sb 12is much smaller than in PrOs 4Sb 12.For PrOs 4Sb 12,the CEF ground state was inferred to be the non-Kramers Γ3doublet carry-ing quadrupole moments,whereas the ground state for PrRu 4Sb 12to be the Γ1singlet.3,9Recently,however,there are several reports that are consistent with the CEF ground state for PrOs 4Sb 12being the Γ1singlet.10,11,12On the comparison in T c with the La compounds,the two compounds have different trend;T c for PrOs 4Sb 12is2 higher than that for La compounds,which is unusual ifwe take into account that PrOs4Sb12contains the mag-netic element Pr ion.These remarkable differences in theunderlying CEF level scheme and hence electronic andSC characteristics between PrOs4Sb12and PrRu4Sb12may be ascribed to an intimate change in the hybridiza-tion strength of Pr-4f state with conduction electronscomprising of respective Os4Sb12-and Ru4Sb12-cage.Inthis context,it is needed that further light is shed uponthe SC and electronic characteristics in the Pr-based su-perconductors.In this paper,we report the normal and SC proper-ties in thefilled-skutterudite compound PrRu4Sb12and LaRu4Sb12via the measurements of NQR frequencyνQand nuclear-spin-lattice-relaxation time T1of Sb nuclei. Single crystals of PrRu4Sb12and LaRu4Sb12were grown by the Sb-flux method.3The observed dHvA os-cillations in both compounds confirm the high quality of the samples.13Measurement of ac-susceptibility con-firmed the SC transitions at T c= 1.3K and3.5Kfor PrRu4Sb12and LaRu4Sb12,respectively.The single crystal was crushed into powder for Sb-NQR measure-ment.The121,123Sb-NQR measurements were performed using the conventional saturation-recovery method atzerofield(H=0).The NQR-T1measurement was car-ried out using the NQR transition2νQ at the T range ofT=0.24K-240K using a He3-He4dilution refrigerator. Fig.1(a)displays the121,123Sb-NQR spectra at4.2K. Sb nuclei has two isotopes121Sb and123Sb.The respec-tive nuclear spin I=5/2(121Sb)and7/2(123Sb)have natural abundance57.3and42.7%,and nuclear gyromag-netic ratioγN=10.189and5.5175[MHz/T],giving rise to two and three NQR transitions,respectively.Fig.1(b)indicates the T dependencies ofνQ(T)derived from the 123Sb-2νQ transition in PrRu4Sb12and LaRu4Sb12.The inset indicatesδνQ(T)=νQ(T)P r−νQ(T)La,which sub-tracts the common effect due to lattice expansion in theboth compounds.νQ(T)reveals a progressive increase upon cooling below T∼70K,which is considered to bedue to the CEF splitting.Note,as shown in Fig.1(c),that theδνQ(T)=νQ(T)P r−νQ(T)La in PrOs4Sb12 was observed to be increased below a temperature com-parable to the CEF splitting∆CEF∼10K between the ground state and thefirst excited state.From this com-parison,∆CEF∼70K is expected in PrRu4Sb12.This is almost consistent with the analysis of susceptibility and resistivity.3,9Fig.2presents the T dependencies of(1/T1T)for PrRu4Sb12and LaRu4Sb12.In the normal state,T1 reveals a Korringa relation(1/T1T)P r=1.73(s·K)−1 for PrRu4Sb12,being comparable to(1/T1T)La= 1.2(s·K)−1for LaRu4Sb12.The1/T1T=w de-viates at temperatures higher than∼30K in PrRu4Sb12. Since such a deviation is seen in LaRu4Sb12above∼25K as well,these deviations are not derived by the presence of Pr3+ions,but may be ascribed to a conduction-band derived effect inherent to thefilled-skutterudite struc-ture.In thefilled-skutterudite structure,a Pr atomTemperature (K)RF(MHz)Temperature (K)RF(MHz)Frequency (MHz)Intensity(arb.units)FIG.1:(a)121Sb-and123Sb-NQR spectra in PrRu4Sb12.(b)The temperature dependence of NQR frequencyνQ for PrRu4Sb12and LaRu4Sb12at the123Sb-2νQ transitions.The inset indicates the Pr-derived contribution inνQ,δνQ= (νQ)P r−(νQ)La.(c)T dependence of NQR frequency for PrOs4Sb12and LaOs4Sb12at123Sb-2νQ transitions.7The In-set indicatesδνQ=(νQ)P r−(νQ)La..forms in a body centered cubic structure,surrounded by a cage of corner-sharing Ru4Sb12octahedra.The cage might begin to stretch with increasing T.This stretch-ing motion of cage may be relevant to the decrease in a value of1/T1T=const.for PrRu4Sb12and LaRu4Sb12 and LaRu4P12.14The measurements of the dHvA ef-fect and the electronic specific heat for PrRu4Sb12and LaRu4Sb12revealed that the mass-renormalization ef-fect in the Fermi liquid state is not so significant in PrRu4Sb12,suggesting that Pr3+-4f2electrons are well localized in PrRu4Sb12.Note that the value of1/T1T is proportional to the square of the density of states N(E F) at the Fermi level.Also,it is scaled to a T-linear elec-tronic contributionγof specific heat,giving rise to the re-lation of(1/T1T)1/2∝γ.Therefore,the change in value of(1/T1T)1/2is directly related to a change of N(E F) in systems.Corroborated by the fact that the value of1/T1T in PrRu4Sb12is not so enhanced than that in1/T 1T (s e c -1K -1)Temperature (K)FIG.2:Temperature dependence of 1/T 1T for PrRu 4Sb 12and LaRu 4Sb 12.Solid lines are the fits calculated based on the weak-coupling s -wave model assuming a size of isotropic gap 2∆/k B T c =3.1and 3.6for PrRu 4Sb 12and LaRu 4Sb 12,respectively.LaRu 4Sb 12with a ratio of [(1/T 1T )P r /(1/T 1T )La ]1/2=1.44,weremark that the Pr +3−4f 2electrons with Γ1singlet as the ground state does not play a vital role for electronic and magnetic properties at low temperatures in PrRu 4Sb 12.1/T 1 (s e c -1)Temperature (K)FIG.3:Temperature dependencies of 1/T 1for PrRu 4Sb 12and PrOs 4Sb 12.7The solid line for PrRu 4Sb 12is the fit based on the weak-coupling s -wave model with 2∆/k B T c =3.1.In the SC state for PrRu 4Sb 12and LaRu 4Sb 12,1/T 1shows a distinct coherence peak,followed by an exponen-tial decrease below T c with an isotropic gap 2∆/k B T c =3.1and 3.6,respectively.These results demonstrate that PrRu 4Sb 12and LaRu 4Sb 12are typical weak-coupling s -wave superconductors.In Fig.3are shown the T depen-dencies of 1/T 1for PrRu 4Sb 12and PrOs 4Sb 12.From the comparison in the normal and SC states,it is clear that remarkable differences arise because the quadrupole de-gree of freedom plays vital role in PrOs 4Sb 12,associated with the Pr +3-4f 2derived non-Kramers doublet.It may indeed argue for Cooper pairing via quadrupolar fluctu-ations.To summarize,the electronic and superconducting properties in the Pr-based filled-skutterudite supercon-ductor PrRu 4Sb 12with T c =1.3K were investi-gated through the measurements of nuclear-quadrupole-resonance (NQR)frequency νQ and nuclear-spin-lattice-relaxation time T 1of Sb nuclei.The T dependence of νQ has revealed the energy scheme of crystal electric field (CEF)of Pr 3+ion that is consistent with an en-ergy separation ∆CEF ∼70K between the ground state and the first-excited level.In the normal state,the Ko-rringa relation of (1/T 1T )P r =const.is valid,revealing a comparable value [(1/T 1T )P r /(1/T 1T )La ]1/2∼ 1.44with (1/T 1T )La for LaRu 4Sb 12.These results are under-stood in terms of the conventional Fermi-liquid picture in which the Pr-4f 2state derives neither magnetic nor quadrupolar degrees of freedom at low temperatures.In the SC state,1/T 1shows a distinct coherence peak just below T c ,followed by an exponential decrease with the value of 2∆/k B T c =3.1.These results demonstrate that PrRu 4Sb 12is a typical weak-coupling s-wave supercon-ductor,in strong contrast with the heavy-fermion super-conductor PrOs 4Sb 12that is in a unconventional strong coupling regime.7The present study on PrRu 4Sb 12high-lights that the Pr-4f 2derived non-magnetic doublet plays a key role for the unconventional electronic and supercon-ducting properties in PrOs 4Sb 12.We thank Y.Aoki and H.Harima for helpful discus-sions.This work was supported by the COE Research (10CE2004)in Grant-in-Aid for Scientific Research from the Ministry of Education,Sport,Science and Culture of Japan.One of the author (H.K.)has been supported by JSPS Research Fellowships for Young Scientists.1C.Sekine,T.Uchiumi,and I.Shirotani,Phys.Rev.Lett.79(1997)3218.2I.Shirotani,T.Uchiumi,K.Ohno,C.Sekine,Y.Nakazawa K.Kanoda,S.Todo and T.Yagi,Phys.Rev.B 56(1997)7866.3N.Takeda,M.Ishikawa,J.Phys.Soc.Jpn.57(2000)868.4E.D.Bauer,N.A.Frederick,P.-C.Ho,V.S.Zapf,and M.B.Maple,Phys.Rev.B 65(2002)100506R.5M.B.Maple,P.-C.Ho,V.S.Zapf,N.A.Frederick,E.D.Bauer,W.M.Yuhasz,F.M.Woodward and J.W.Lynn,J.Phys.Soc.Jpn.71(2002)23-286D.L.Cox,Phys.Rev.Lett.59,(1987)1240.7H.Kotegawa,M.Yogi,Y.Imamura,Y.Kawasaki,G.-q.Zheng,Y.Kitaoka,S.Ohsaki,H.Sugawara,Y.Aoki,andH.Sato,Phys.Rev.Lett.70,(2003)027001-1.8H.Sugawara,S.Osaki,S.R.Saha,Y.Aoki,H.Sato,Y.Inada,H.Shishido,R.Settai,Y.¯Onuki,H.Harima and K.Oikawa,Phys.Rev.B66(2002)220504R.9K.Abe,H.Sato,T.D.Matsuda,T.Namiki,H.Sugawara,Y.Aoki,J.Phys.:Condens.Matter14(2002)11757-11768.10Y.Aoki,T.Namiki,S,Ohsaki,S.R.Saha,H.Sugawara,H.Sato,J.Phys.Soc.Jpn.71(2002)2098-210111M.Kohgi,et al.,appear in J.Phys.Soc.Jpn.12T.Tayama et al.,unpublished.13T.D.Matsuda,K.Abe,F.Watanuki,H.Sugawara,Y.Aoki,H.Sato,Y.Inada,R.Settai and Y.¯Onuki,PhysicaB312-313(2002)832-833.14K.Fujiwara,K.Ishihara,K.Miyoshi,J.Takeuchi, C.Sekine,I.Sirotani,Physica B281-282(2000)296-297.TABLE I:Comparison of the superconducting critical tem-perature T c,superconducting specific heat jump∆C dividedby T C(∆C/T C).Sommerfeld coefficient,and effective massm∗c in RT4Sb12(R=La,Pr,T=Ru,Os).8T C(K) 1.850.74 1.3 3.58∆C/T C(mJ/K2mol)5008411082 Sommerfeld coefficient(mJ/K2mol)350∼75036,565937 m∗c/m0forγ-branch7.6 2.8 1.6 1.4。