Soil Contributions to Blasting Effects from Case Histories
AnEmpiricalSoilLossEquation-tucson.ars.ag.gov

An Empirical Soil Loss EquationLiu Baoyuan, Zhang Keli and Xie YunDepartment of Resources and Environmental Sciences, Beijing Normal University, Key Laboratory of Environmental Change and Natural Disaster, the Ministry of Education of China,Beijing 100875, PR ChinaAbstract: A model was developed for estimating average annual soil loss by water on hillslope for cropland, which is called Chines Soil Loss Equation (CSLE). Six factors causing soil loss were evaluated based on soil loss data collected from experiment stations covering most regions of China and modified to the scale of Chinese unit plot defined. The model uses an empirical multiplicative equation, A=RKLSBET, for predicting interrill erosion from farmland under different soil conservation practices. Rainfall erosivity (R) was the product of rainfall amount and maximum intensity of 10min, and also was estimated by using daily rainfall data. The value of soil erodibility (K), the average soil loss of unit plot per rainfall erosivity, for 6 main soil types was calculated based on the data measured from unit plots and other data modified to the unit plot level. The method of calculating Kfrom soil survey data for regions without measured data was given. The slope length and steepness factors was calculated by using the equations in USLE if slope steepness is less than 11 degree, otherwise the steepness factor was evaluated by using a new seep slope equation based on the analysis of measured soil loss data from steep slope plots within China.According to the soil and water conservation practices in China, the values of bio-control, engineering-control, and tillage factors were estimated.Keywords: Chines soil loss equation, soil loss, unit plot1 IntroductionSoil loss equation is to predict soil loss by using mathematical methods to evaluate factors causing soil erosion. It is an effective tool for assessing soil conservation measures and making land use plans. Universal soil loss equation is an empirical equation developed during 1950’s that had been applied for natural resources inventory successfully in the US and revised in 1990’s. From 1980’s the process-based models for prediction of soil loss have been studied though the world, such as WEPP (Water Erosion Predict Project, Nearing et al., 1989), GUEST (Griffith University Erosion System Template, Misra and Rose, 1996), EUROSEM (the European Soil Erosion Model Morgan etal., 1998) and LISEM (Limburg Soil Erosion Model, De Roo and Wesseling, 1996). There have been many studies on soil erosion models and related experiments since 1940's, but the models were limited in local levels and difficult to expand to broad regions due to data collected without universal standard. So far there is not any soil loss equations that could be applied through China with minor errors. The objective of this study is to develop a soil loss equation used within China based on measured data from Chinese unit plots and data from many plots modified to Chinese unit plot, which is called Chinese soil loss equation (CSLE).2 Model descriptionSoil loss is a process of soil particle detachment by raindrops and then transported by runoff from the rainfall. Many factors like soil physical characteristics slope features, land surface cover etc. will influence soil loss amount, but they have interactions. It is necessary to distinct their effects on soil loss mathematically and to evaluate them on the same scale in order to improve the accuracy of the model. Unit plot is such a good method to solve the problem. The normalized data covering the China by modified to unit plot supported the development of Chines soil loss equation. In addition,22 two features of soil erosion in China are distinctive and should be considered in the equation. One issoil erosion with steep slope, and the other is the systematical practices for soil conservation during thelong history of combating soil erosion, which could be classified as biological-control, engineering-control and tillage measures. So the Chinese soil loss equation was express as followsafter the analysis of data collected from most regions of ChinaA = RKSLBET (1)where A is annual average soil loss (t/ha), R is rainfall erosivity (MJ mm/(h ha y)), K is soilerosibility (t ha h/(ha MJ mm y)), S and L are dimensionless slope steepness and slope lengthfactors, B , E , and T are dimensionless factors of biological-control, engineering-control, and tillagepractices respectively. The dimensionless factors of slope and soil conservation measures were defined as the ratio of soil loss from unit plot to actual plot with aimed factor changed but the samesizes of other factors as unit plot. Chinese soil loss equation is to predict annual average soil lossfrom slope cropland under different soil conservation practices.To evaluated factors in the equation, about 1841 plot-year data were analyzed. Of these, 214plot-year data from 12 plots and 1143 rainfall events from 14 weather stations were used to evaluaterainfall erosivity. The Chinese unit plot was determined by analyzing 384 plot size data, and about200 plot-year data from 12 plots modified to unit plot were used to evaluate erodibility for 6 types ofsoil. About 30 plot-year data from steep plots modified to unit plot was used to establish the steepslope factor equation. Other plot data was used to calculate the values of biological-control, engineering-control and tillage factors.3 Factor calculations for the equation3.1 Rainfall erosivity (R )A threshold for erosive rainfall of 12mm was estimated, close to that suggested by Wischmeierand Smith (1978), 12.7mm. After comprehensive considering the accuracy of rainfall erosivity, thedata availability and calculation simplicity, the rainfall index of a rainfall event for Chinese soil losserosion was defined. It is the product of rainfall amount (P ) and its maximum 10-min intensity (I 10),and the relationship between PI 10 and the universal rainfall index EI 30 was also estimated as follows:EI300.1773PI 10 R 20.902 (2)where E is the total energy for a rainfall event (MJ/ha), I 30 and I 10 are the rainfall maximum 30min and10min intensities respectively (mm/hr), P is the rainfall amount (mm). The annual rainfall erosivityis the sum of PI 10 for total rainfalls through the year. Actually, it is also difficult to get the rainfallevent data. To apply the weather data from weather stations covering the China, an equation functionfor estimating half-month rainfall erosivity by using daily rainfall data was developed.1010.184(n hm d d i i R P ==∑)IR 20.973 (3)where R hm is the rainfall erosivity for half-month (MJ mm/h ha), P d is the daily rainfall amount (mm)and I 10d is the daily maximum 10min rainfall intensivity (mm/h). I =1, …, n is the rainfall days withina half-month. If there is no I 10d available, R hm was also calculated by using only daily rainfall amount.1()n hm d i i R P βα==∑ (4)where α and β are fitted coefficients and other variables had the same meaning as above.Seasonal rainfall erosivity distribution could be estimated by the sum of R hm . To plot Chineseisoerodent map for estimation or interpolation of local values of average annual rainfall erosivity in23 any place, the empirical relationships by using different rainfall available data were estimated (not listed). Users can choose different equations to calculate average annual rainfall erosivity according to the data availability.3.2 Soil erodibility (K)Soil erodibility is defined as soil loss from unit plot with 22.1m long and 9% slope degree per rainfall erosion index unit (Olson and Wischmeier, 1963). Different from the US, much of soil loss was from steep slope in China. So the Chinese unit plot was defined as a 20m long, 5m wide and 15°degree slope plot with continuously in a clean-tilled fallow condition and tillage performed upslope and downslope. The suggestion of Chinese unit plot made data measured be used to evaluate K values as much as possible without large errors. Because 15° is the middle values for most plots in China. Modified data measured from both plots of less than 15° and larger than 15° to a unit plot had the relative minimum errors.Based on the K defination and Chines unit plot, soil erodibility for 6 main soil types in China was estimated. For example, the values of K for loess were 0.61, 0.33, and 0.44 t ha h/(ha MJ mm) in Zizhou, Ansai, and Lishi in Loess Plateau of China.3.3 Slope length (L) and slope steepness (S) factorsTopography is an important factor affecting soil erosion. It is significant to quantitatively evaluate the effects of topography on erosion for predicting soil loss. The effects of topography on erosion includes slope length and steepness in terms of soil-loss estimation. In soil loss equation, factors of slope length and steepenss were cited with dimensionless values. They are values of the ratio of the soil loss from the plot with actual slope steepness or slope length to that from the unit plot.The relationship between slope length and soil loss has been studied from field or lab data for a long time. Many studies showed that the soil loss per area is proportional to some power of slope length except that the values of the exponent are slightly different. For example, Zingg (1940) derived a value of 0.6 for the slope-length exponent. Musgrave (1947) used 0.35. USLE (Universal Soil Loss Equation ) published in 1965 suggested the values of 0.6 and 0.3 respectively for slopes steeper than 10% and very long slopes, and 0.5 for other conditions. In 1978, the USLE (Wischmeier and Smith, 1978) adjusted the exponent values for different cases, 0.5 for 5% slope or more, 0.4 for slopes between 3.5% and 4.5%, 0.3 for slopes between 1% and 3%, and 0.2 for slopes less than 1%. Revised USLE (RUSLE) published in 1997 used a continuous function of slope gradient for calculating slope-length exponent.Soil erosion from the steep slope is serious in China. How slope length influences soil loss on steep slopes needs further studies. For this end, the relationship between slope length and soil loss on steep slopes was examined based on the plot data obtained at Suide, Ansai, and Zizhou on the loess plateau of China and modified to the unit plot. The results indicated that the slope-length equation in the RUSLE could not be used for soil loss prediction under the steep slope conditions. The equation for calculating soil length factor in the USLE published in 1978 could be applied into China:22.13mLλ=(5)where λ is slope-length (m), m is the slope length exponent.Slope gradient is another topographical factor affecting soil erosion. Most studies have shown that the relation of soil loss to gradient may be expressed as some exponential function or quadratic polynomial. Zingg (1940) concluded that soil loss varies as the 1.4 power of percent slope, and Musgrave (1947) recommended the use of 1.35. Based on a substantial number of field data, Wischmeier and Smith (1965) derived a slope-gradient equation expressed as quadratic polynomial function of gradient percent. Having analyzed the data assembled from plots under natural and simulated rainfall, McCool et al., (1987) found that soil loss increased more rapidly from the slopes24 steeper than 5° than that from slopes less than 5°, and he recommended two different slope steepnessfactor equations for different ranges of slopes:S =10.8sin θ + 0.03 θ 5° (6-1)S =16.8 sin θ – 0.5 θ > 5°(6-2) These equations were established based on soil loss data from gentle slopes, and have not beentested for steep slope conditions. We used soil loss plot data from Suide, Ansai, and Tianshui on theloess plateau of China to test the equations. The results showed that great errors were produced whenusing equations suggested by McCool et al ., (1987) for predict soil loss from slopes steeper than 10°. After the slope degree was larger than 10°, soil loss from steep slopes increased ripedly. Based theregression analysis of our data, am equation to calculate slope steepness factor for seep slopes wasdeveloped:S =21.91 sin θ – 0.96 θ10°(6-3)So in Chinese soil loss equation, slope steepness factor could be estimated by using equation (6-1)to (6-3) under different slope conditions3.4 Bilogical-control (B ), engineering-control (E ), and tillage (T ) factorsDuring the development of the historical agriculture traditions in China, the systematical practicesfor soil and water conservation formed. They could be divided into three categories: biological-control, Engineering-control and tillage measures. Biological-control practices include theforest or grass plantation for reducing runoff and soil loss. Engineering-control practices refer to thechanges of topography to reduce runoff and soil loss by engineering construction like terrace, check-dams. Tillage practices are the measures taken by farmland equipment. The difference between engineering and tillage is that the latter does not change the topography and is only applied onthe farmland.Table 1 Estimated values of biological-control factor for cropsSeedbed Establishment Development Maturing crop Growing season Annual averageBuckwheat 0.71 0.54 0.19 0.21 0.74 0.74 Potato 1.00 0.53 0.47 0.30 0.47 0.50 Millet 1.00 0.57 0.52 0.52 0.53 0.55 Soybean 1.00 0.92 0.56 0.46 0.51 0.53 Winter wheat 1.00 0.17 0.23Maize intercropping with soybean1.00 0.40 0.26 0.03 0.28 Hyacinth Dolichos 1.00 0.70 0.46 0.57Table 2 Estimated values for factors of woodland and grassland vegetationSophora Korshinsk Peashrub Seabuckthorn Seabuckthorn & PoplarSeabuckthorn & Chinese Pine Erect Milkvetch Sainfoin Alfalfa First year Sweetclover Second year Sweetclover0.004 0.054 0.083 0.144 0.164 0.067 0.160 0.256 0.377 0.08325Many studies gave the B values for different biologic measures in China, but they were not from the universal calculated methods and could not be used directly in soil loss equation. Based on the defination of B values, the ratio of soil loss from plots with some biological-control practice to that from unit plot, we calculated B values for some types of biological-control practices (Table 1). Some values for typical engineering-control and tillage measures in China were summarized (not listed).References[1] Nearing, M.A., G.R. Foster, L.J. Lane, and S.C. Finkner. A process-based soil erosion modelfor USDA-water erosion prediction project technology. Transactions of The ASAE, 1989, 32(5):1587-1593.[2] Misra R K, Rose C W. Application and sensitivity analysis of process -based erosionmodel—GUEST[J]. European Journal Soil Science. 1996,10:593-604.[3] Morgan R P C, Quinton J N, Smith R E, et al., The European soil erosion model (EUROSEM): Adynamic approach for predicting sediment transport from fields and small catchments[J]. Earth Surface Processes and Landforms, 1998,23:527-544.[4] De Roo A P J. The LISEM project: an introduction. Hydrological Processes. 1996, vol.10:1021-1025.[5] Wischmeier W H, Smith D D. Predicting rainfall erosion losses[R]. USDA AgriculturalHandbook No.537. 1978.[6] Olson,T.C., and Wischmeier,W.H. 1963. Soil erodibility evaluations for soils on the runoff anderosion stations. Soil Science Society of American Proceedings 27(5):590-592.[7] Zingg A W. Degree and length of land slope as it affects soil loss in runoff[J]. AgriculturalEngineering, 1940,21: 59-64.[8] Musgrave G W. The quantitative evaluation of factors in water erosion A first approximation[J].Journal Soil and Water Cons, 1947, 2:133-138.[9] Renard K G,Foster G R,Weesies G A et al., RUSLE―A guide to conservation planning with therevised universal soil loss equation[R]. USDA Agricultural Handbook No.703. 1997.[10] McCool, D. K. Brown, L.C., Foster, G. R., et al., Revised slope steepness factor for theuniversal soil loss equation. TRANSACTIONS of the ASAE, 1987, 30(5): 1387-1396.。
土壤侵蚀敏感度计算

土壤侵蚀敏感度计算英文回答:Soil erosion sensitivity is a measure of the susceptibility of a soil to erosion. It is determined by a number of factors, including the soil's texture, structure, organic matter content, and slope. Soils with a high erosion sensitivity are more likely to be eroded by wind and water, while soils with a low erosion sensitivity are less likely to be eroded.There are a number of different methods for calculating soil erosion sensitivity. One common method is the Revised Universal Soil Loss Equation (RUSLE), which is used to estimate the average annual soil loss from a given area of land. RUSLE takes into account the soil's texture, structure, organic matter content, slope, and rainfall erosivity.Another common method for calculating soil erosionsensitivity is the Soil Erosion Potential (SEP) index, which is used to estimate the potential for soil erosion on a given area of land. SEP takes into account the soil's texture, structure, organic matter content, slope, and land use.Soil erosion sensitivity is an important factor to consider when planning land use activities. Areas with a high erosion sensitivity should be managed carefully to prevent soil erosion. This can be done by using conservation practices such as terraces, contour farming, and cover crops.中文回答:土壤侵蚀敏感性是指土壤对侵蚀的敏感程度。
Soil Contamination EP博士讲座

The case for China - Overall
• Soil pollution comes mainly from mining and industrial activity. China’s 30 years of rapid economic growth have inevitably caused many environmental problems. Europe and the US saw the same thing during their periods of quick growth.
• B-horizon /(subsoil): less organic material & fewer organisms than A- horizon.
• C-horizon: consists of broken-up bedrock, does not contain any organic materials. Chemical composition helps to determine pH of soil & also influences soil’s rate of water absorption & retention.
The contaminated soil reached about 10.500.000 hectares(公顷). The soil contaminated resulting from sewage irrigation was 2.275.000 hectares, and the soil stockpiled and ruined by solid waste was 140.000 hectares. These covered 10% of the total cultivated area. As different kinds of industrial wastewater, exhaust gas, livestock manures, sewage irrigation and sludge farm application have all become the sources of heavy metals.
市政垃圾堆肥对巴西土壤的化学效应

Bioresource Technology 98 (2007) 525–5330960-8524/$ - see front matter © 2006 Elsevier Ltd. All rights reserved.doi:10.1016/j.biortech.2006.02.025Composted municipal waste e V ects on chemical propertiesof a Brazilian soilD.V. Pérez a,¤, S. Alcantara b , C.C. Ribeiro b , R.E. Pereira b , G.C. Fontes b , M.A. Wasserman c ,T.C. Venezuela d , N.A. Meneguelli a , J.R. de Macedo a , C.A.A. Barradas eaEmbrapa-Solos, R. Jardim Botânico, 1024, Rio de Janeiro (RJ), BrazilbInstituto de Química, UFRJ, Av. Brig. Trompovsky, s/no, Cidade Universitária, Rio de Janeiro (RJ), Brazil cInstituto de Radioproteção e Dosimetria/CNEN. Av. Salvador Allende s/no, Recreio, Rio de Janeiro (RJ), BrazildFiocruz/ENSP, R. Leopoldo Bulhões, 1480, Rio de Janeiro (RJ), 21041-210, Brazil ePESAGRO-RIO, R. Euclydes Solon Pontes no 30, Nova Friburgo (RJ), 28.625-020, BrazilReceived 4 January 2004; received in revised form 12 January 2006; accepted 7 February 2006Available online 31 March 2006AbstractThe spread of composted municipal waste (CMW) on land can be used for sustainable crop production. Nevertheless, heavy metals availability may be a problem. Therefore, the main objective of this study was to assess the impact of CMW disposal on heavy metal accu-mulation in soil and plants. The treatments consisted of an untreated plot (control) and four rates of CMW application. All plots were cultivated in succession of carrot, cauli X ower, sweet corn, and radish. Cu and Pb signi W cantly accumulated in the topsoil (0–5cm) with a similar pattern in the depths of 5–10cm and 10–20cm. Cauli X ower, for Fe and Cu, and radish, for Pb and Cu, had their tissue analysis sig-ni W cantly a V ected due to the increasing rates of application of CMW. Nevertheless, the levels of accumulation in both, soil and plant, are within permissible limits. The evidences provided by this experiment indicated that heavy metals are less likely to cause problems for the estimation of CMW loadings to Brazilian agricultural land.© 2006 Elsevier Ltd. All rights reserved.Keywords:Municipal waste; Biosolid; Heavy metals; Soil properties1. IntroductionMunicipal solid waste has been a serious environmental problem for many cities in developing countries (Abdel-Sabour and Abo El-Seoud, 1996; Soumaré et al., 2003). For instance, Rio de Janeiro, the second largest city in Brazil,has shown over the last years a signi W cant increase of solid waste handling. From 1995 to 2002, the total waste col-lected and the average waste collected per day increased from 2.6 to 3.6 million tons and 7.0 to 9.9 thousand tons,respectively.In spite of the fact that there are many kinds of W nal disposal available, the agricultural practice of amending soils with composted municipal waste (CMW) has received worldwide attention. This compost is rich in organic matter and its use may improve soil fertility and physical proper-ties (He et al., 1992; Oshins, 1995; Anikwe and Nwobodo,2002). However, repeated compost application can lead to accumulation of trace metals in soils that could eventually contaminate human and other animal food chains (Garcia et al., 1992; Pinamonti et al., 1997; National Research Council, 2003).There are currently no standards to evaluate compost quality in Brazil. Only Paraná and São Paulo states have set up guidelines based on the criteria for sludge (Sanepar,1997; CE TE SB, 2001). Hence, there is a need to develop*Corresponding author.E-mail address: daniel@cnps.embrapa.br (D.V. Pérez).526 D.V. Pérez et al. / Bioresource Technology 98 (2007) 525–533general standards for land application of urban waste. Our objectives were to determine: (i) the e V ect of CMW applica-tion on the heavy metal composition of four vegetable crops and (ii) the residual e V ects of this soil amendment on chemical properties of a Brazilian soil.2. MethodsAll the composted municipal waste used during this study was taken from the largest treatment plant (Caju) of Rio de Janeiro State, and it was applied from 1995 to 1997to a soil (Dystrochrept) located in Nova Friburgo County (22°15ЈS and 42°45ЈW), a representative mountain area of intensive vegetable production. The Köppen climate classi-W cation is Cwb with mean annual temperature of 18°C and mean annual precipitation of 1431mm. In Caju plant, recy-clable materials such as paper, glass and aluminum cans are manually removed early in the composting process. The remaining material is ground and the ferrous materials are separated using an electromagnet. The remaining organic waste is sieved and arranged into rows of long piles, and aerated by turning the pile periodically with mechanical means. Generally, 77–90 days are necessary to complete the composting.Some characteristics of the soil used in this study are presented in Table 1. The experimental W eld design was a randomized complete block, consisting of an untreated plot (control) and four rates of CMW surface application (12.5,25, 50, 100t ha ¡1, wet basis) and four blocks. No inorganic fertilizer was applied. Irrigation was also applied so as to keep soil moisture in the range of W eld capacity. Each plot had a total area of 4.0£2.4m 2 separated in all directions by a border of 1m. All plots were cultivated with the following crop succession: carrot, planted on November 22nd 1995and harvested on April 2nd 1996; cauli X ower, planted on May 23rd 1996 and harvested on September 5th 1996;sweet corn, planted on December 10th 1996 and harvested on April 10th 1997; and radish, planted on August 20th 1997 and harvested on October 21st 1997. These species were selected due to the fact that they present di V erent edi-ble plant parts (root, X ower, seed) and, consequently, di V er-ent nutrient uptake paths (Farago, 1994). They are also sensitive crops for di V erent metal toxicities (Alloway, 1990;Fergusson, 1990; Kabata-Pendias and Pendias, 1992).Rotary tilling was used in order to prepare the soil since it mixes the upper layers of soil rather than completely turn-ing the soil over. In the present study, tillage went to the W rst 15cm of the soil. Moreover, the direction of the rotary tilling was inverted after each cultivation so as to avoidplot-to-plot contamination. The compost treatments were super W cially applied in the same day of planting. Hence, a total amount of 50, 100, 200, and 400t ha ¡1 CMW was applied during the experiment. After the W rst year of the last CMW spread (1998), soil samples were collected from four depths (0–5, 5–10, 10–20, 20–40cm). The samples were dried in a forced air oven at 40°C, passed thorough a 2-mm sieve and stored in a polyethylene bag. Vegetable edible parts were rinsed with distilled water, after that they were dried at 70°C and ground in a stainless steel Wiley mill in order to pass a 1mm sieve. Ground samples were stored at room temperature in acid-washed polyethylene containers.Aqua regia (HCl/HNO 3, 3:1) extraction is used in order to evaluate anthropogenic inputs of heavy metals and it is rather e Y cient (Hani, 1996; Walter and Cuevas, 1999;Scancar et al., 2000). Hence, this method was applied for soil and compost evaluation of Fe, Mn, Zn, Cu, Cd, Cr, Pb,and Ni. Therefore, considering the accumulation of some metals observed in the 0–5cm, a sequential extraction method (Wasserman et al., 2005; Table 2) was used in order to specify the types of metal associations in soil.Dried plant samples were digested with a nitric/perchloric acid mixture. The content of the selected metals was ana-lyzed by ICP-OE S (Perkin–E lmer OPTIMA 3000, Nor-walk, CT, USA). Duplicate analyses of each soil and plant extractions were performed throughout the work as well.The humic fractions of the soil were sequentially extracted according to Kononova and Bel’Chikova (Sastri-ques, 1982). In short, the free fulvic acids (not bound) were obtained by shaking 20–40g of soil (air dried, 2mm-sieved)Table 1Some physical and chemical characteristics a of the soil used in the experimentaDetermined according to the procedures of EMBRAPA (1997).Horizon Depth (cm)Clay (g/kg)pH (H 2O)Ca 2++Mg 2+ (cmol c /kg)K + (cmol c /kg)Al 3+ (cmol c /kg)CEC (cmol c /kg)P (mg kg ¡1) C (g/kg)N (g/kg)Ap 0–20330 6.0 3.6 1.10.840.011.125114.80.22Bi120–45390 5.20.80.120.612.03418.00.25Bi245–70380 5.10.70.070.69.03418.40.25Table 2Procedure of the sequential extraction scheme used to fractionate heavy metals in soil samples FractionSequence of extraction(F1)HAc (2M)+NaAc (2M) 1:1; pH 4.7Exchangeable +carbonate bound Room temperature/16h(F2)NH 2OH ·HCl (0,1M); pH 2 (HNO 3)Fe & Mn oxide bound Room temperature/16h (F3)H 2O 2 (30%)+HNO 3(0.02M)+NH 4Ac (1M)Room temperature/16h Organically bound (F4)NaOH (0.1M); pH 12Al oxide +strong Fe &Mn oxide bound Room temperature/16h (F5)Aqua regia (HNO 3/HCl; 1:3)Residual50°C/30minD.V. Pérez et al. / Bioresource Technology 98 (2007) 525–533527with 200mL H 3PO 4 2M for 30min. After that, the sample was centrifuged at 1000rpm for 30min and it was also W ltered. This procedure was repeated 3 times. The residual soil was washed 3 times with demineralized water and it was shaken with 200mL Na 4P 2O 7 0.1M +NaOH 0.1M for 4 hours. Following centrifugation (3000rpm/30min) the supernatant solution (humic +fulvic acids) was separated.This procedure was repeated 3 times as well. The residual soil was used so as to determine the C content of the humin fraction. An aliquot of the supernatant was acidi W ed with H 2SO 4 to pH 1.0 and, then, centrifuged (1500rpm/5min)in order to separate the precipitated humic acid from the supernatant fulvic acid. The carbon content of each frac-tion was determined by dichromate oxidation.Suprapur acids (Merck, Darmstadt, Germany) and ultrapure water (Barnstead Ultrapure Water System,Dubuque, IA, USA) were used in all laboratory procedures.All containers were soaked in 10% HNO 3 and thoroughly rinsed in demineralized water before being used.An analysis of variance was used in order to test sig-ni W cance (P <0.05) of treatment e V ects and Tukey’s test (P <0.05) was used so as to compare the means. All stat-istical analyses were performed using Statistical Analysis System (SAS, 1999).3. Results and discussion3.1. Chemical composition of the CMWIn spite of the fact that there are currently no Brazilian standards in order to evaluate compost quality, the results (Table 3) agree well with the scarce data of chemical analy-sis of Brazilian CMW. Grossi (1993), during 1990 and 1991,analyzed 61 samples of CMW from 16 Brazilian cities. She found a large range of heavy metal concentrations varying with the maturity degree of the compost (Table 4). Based on it, our results match most of the ranges in heavy metals which were related to the mature compost that we used (Tables 3 and 4). Our results also match the ranges of heavy metals found by Cravo et al. (1998) during their study of the compost produced by six Brazilian waste plant treat-ments (Tables 3 and 4). Considering the main international legislation on the disposal of biosolids in agriculture (USE PA 40 CFR Part 503 and Council Directive 86/278/EEC), we realized that, all the metal contents determined in the present study were under the regulation limits (Tables 3and 4). Notwithstanding, some countries in E urope have more restricted laws (Table 4). In that case, the CMW employed in the present work failed to match the limitTable 3Some chemical analyses of the CMW used as amendment for the cultivation of the four vegetables in BrazilaWet basis. Relative standard deviation lower than 10% for all analyses.Vegetable crop pH Moisture (g kg ¡1)a C(g kg ¡1)N (g kg ¡1)C:N Fe (£103)(mg kg ¡1)Mn (mg kg ¡1)Cu (mg kg ¡1)Cd (mg kg ¡1)Co (mg kg ¡1)Cr (mg kg ¡1)Ni (mg kg ¡1)Pb (mg kg ¡1)Carrot7.7407.2158.715.21012.6240.2384.4 2.0 2.064.128.8189.0Cauli X ower 7.7484.6162.015.4119.3198.2170.5 1.4 1.656.217.1151.0Sweet corn 7.8440.8130.916.289.6203.0248.0 1.5 1.854.029.1153.0Radish7.7173.8154.717.8921.6336.2119.8 1.7 2.541.020.384.0Table 4Some chemical analyses of the CMW produced in Brazilian cities compared with various international regulation limits for biosolids disposal in agricul-ture a Cravo et al. (1998), mean and standard deviation, in parenthesis.b Grossi (1993) for mature compost.c Grossi (1993) for semi-mature compost.d Grossi (1993) for raw compost.e USEPA (1994) for Exceptional Quality biosolid.f Long (2001) for grade B biosolid.gEuropa (2005).Source Fe (£103) (mg kg ¡1dry-matter)Mn (mg kg ¡1dry-matter)Cu (mg kg ¡1dry-matter)Cd (mg kg ¡1dry-matter)Co (mg kg ¡1dry-matter)Cr (mg kg ¡1dry-matter)Ni (mg kg ¡1dry-matter)Pb (mg kg ¡1dry-matter)Brazil a23.3304229 2.810.889.832238.9(13.7)(132)(266)(1.7)(4.9)(45.1)(27)(166.4)Brazil b 11–23–61–2330.1–0.576–10420–3144–342Brazil c 103–572–72–29910.3–12.860–27819–7857–1272Brazil d 88–252–64–19700.7–4.533–13317–3844–274USA e––150039–1200420300Australia f ––250020500–3000270420EuropeanCommunity g ––1000–175020–40––300–400750–1200France g ––1000151000200800Germany g ––80010900200900Netherlands g ––75 1.257530100Sweden g600210050100528 D.V. Pérez et al. / Bioresource Technology 98 (2007) 525–533concentrations of Cu, Cd and Pb related to The Nether-lands and Pb to Sweden laws. Concerning only the four applications of the CMW used in the experiment, we have observed that there were some variations of trace metals content (Table 3). However, they were probably related to seasonal variations in raw input of the collected municipal waste.The contents of trace metals found in the compost were much higher than in the agricultural soil used (Table 5) for Cu, Cr, and Pb. However, the concentrations of Fe, Mn,Cd, and Co were higher in the soil and the results for Ni varied. The outcomes concerning heavy metal accumula-tion in soil will be discussed in a coming section.No negative e V ect was observed due to the increasing application of compost rates in the yield of the four culti-vated vegetables (Fig.1). Hence, the CMW used seemed to have no phytotoxicity e V ect.3.2. Plant analysisNo Cd was detected in the analyzed tissues (Table 6).Depending upon the tissue, there were some other elements,among those determined, which were below limit detection.In that case, they were not shown (Table 6). The e V ect of the compost on the uptake of trace metals analyzed is apparently insigni W cant for carrot and sweet corn. For cau-li X ower, Fe and Cu uptakes decreased after the application of higher rates of CMW. However, Cu and Pb uptakes for radish have increased, although, in the case of Cu, it occurred only with the highest amount of compost applica-tion (Table 6).When compared to other studies dealing with metal absorption from crops amended with CMW, our resultsshow a di V erent trend. Fritz and Venter (1988) studied, in a greenhouse experiment, the heavy metal uptake behavior of lettuce, spinach, carrot, radish, bush bean, and tomato, all of them were grown successively. Their results of leaf analy-sis, averaged over all species, indicated that Zn, Cu, and Pb increased signi W cantly due to the higher compost rates used.Cd, Cr, and Ni did not show any signi W cant variation. Root crops had shown a signi W cant increase of Zn. However,only carrot roots exhibited a considerable increase of Pb and Cr contents. Fruit crops have shown signi W cant di V er-ences for Zn, Ni, Cd, and Cr, but only for the intermediate CMW doses. Costa et al. (1994) observed, in a greenhouse experiment with lettuce, increased Zn, Cu, Cd, and Pb leaf contents with increasing compost rates. Abdel-Sabour and Abo El-Seoud (1996) observed, in sesame seeds, an increase of Pb and Cd concentrations with CMW treatments in rela-tion to the control. Pinamonti et al. (1997) found a signi W -cant increase in Ni and Cr in the leaves and fruits of an apple orchard, but only for the highest doses of CMW.Costa et al. (1997) observed in a greenhouse experiment that compost application had enhanced Zn, Cu, and Cd accumulation on dry-matter of carrot leaves and roots.The marked heterogeneity among the studies cited above and also to those found in the present work is probably related to the fact that they were dealing with di V erent plant species under di V erent and speci W c soil conditions (Fritz and Venter, 1988; Kabata-Pendias and Pendias,1992; Pais and Jones, 1997).Based on data given in Table 6 and according to Kabata-Pendias and Pendias (1992), it is possible to a Y rm that there was no metal concentration above the level considered critical for plant tissues in general. However,our results must be viewed cautiously. The experiment with composted waste endured two years only, and in some treatments a very high rate of CMW (400t ha ¡1) was employed. Consequently, we are not able to state whether any drawback would come out if CMW was used for more than two years, especially when it comes to such high rates of CMW.3.3. Soil analysis3.3.1. “Total” metal concentrationSoil contents and variabilities (Table 7) were within the range considered normal in soils (Kabata-Pendias and Pen-dias, 1992; Pais and Jones, 1997), corroborating the fact that no phytotoxicity e V ect was found by the application ofTable 5Metal concentrations of the soil at the experimental site in Nova Friburgo county (Brazil) before the application of compost waste treatments Relative standard deviation lower than 10% for all analysis.Depth (cm)Mn (mg kg ¡1dry-matter)Fe (£103) (mg kg ¡1dry-matter)Cd (mg kg ¡1dry-matter)Co (mg kg ¡1dry-matter)Cr (mg kg ¡1dry-matter)Cu (mg kg ¡1dry-matter)Ni (mg kg ¡1dry-matter)Pb (mg kg ¡1dry-matter)0–5401.040.7 2.418.323.517.820.323.95–10388.639.7 2.319.022.017.721.722.710–20396.641.1 2.121.122.917.221.923.520–40388.530.82.522.122.814.120.314.7D.V. Pérez et al. / Bioresource Technology 98 (2007) 525–533529CMW (Fig.1). The e V ect obtained with the studied treat-ments on the aqua regia (“Total”) heavy metal content,concerning the soil upper layer (0–5cm) can be related to the amounts of each element introduced by the CMW.Hence, the applied composts have led to a great input of Cu, Cr, and Pb in the original soil (Tables 4 and 5), which caused an important increase in the “Total” concentration of these elements in the soil (Table 7). The “Total” Co con-tent has consistently decreased in virtue of an increase in the amount of CMW (Table 7), due to the fact that the Co content of CMW was lower than the soil Co concentration (Tables 4 and 5). In the case of Fe and Mn, although the same pattern of di V erence between soil and CMW concen-trations occurred, similar to the Co, the soil content is in an order of magnitude higher where the dilution by the CMW application is insigni W cant. The concentration of Ni in both the soil and in the CMW did not di V er signi W cantly.As a consequence, there was no signi W cant variation due to CMW application. Cabrera et al. (1989) and Pinamonti et al. (1997) observed that relationship between the soil and CMW heavy metal concentration so as to predict the soil accumulation of these elements.Data for “Total” contents of all studied metals with depth were similar to the corresponding 0–5cm layer, with the exception of the layer below 20cm. That is, Pb and Cu concentrations have increased signi W cantly after CMW application and the levels of the other metals have shownno signi W cant variation. The exception was Cr, since there was no signi W cant di V erence of its content between the 5–10and the 10–20cm layers. However, it is possible to say that numerically, the Cr content increased when higher levels of CMW were applied. Hence, the increase of Pb and Cu concentrations, associated with the higher rates of CMW application, in the layers 0–5cm, 5–10cm and 10–20cm probably resulted from the e V ect of mixing caused by roto-tilling the soil to a depth of 15cm. Data from Co seems to corroborate this. The dilution observed in the 0–5cm depth,caused by the lower concentration of Co present in CMW than in the soil, occurred also in the 5–10cm depth. At the depth of 20–40cm, except for Cr and Ni, most of the metal contents were una V ected by the treatments. The signi W cant di V erence found for Cr at the depth of 20–40cm was consis-tent with the trend observed for 5–10, and 10–20cm layers,although, in that case, it was not statistically signi W cant.The results for Ni showed a slightly low value for the 50t ha ¡1 CMW application, which a V ected the statistical mean test.3.3.2. Sequential extractionBesides soil-to-plant transfer of heavy metals, direct ingestion of soil by humans and animals are important pol-lutant transport pathways to be considered for land appli-cation of biosolids (Ryan and Chaney, 1994; Iskandar and Kirkham, 2001; National Research Council, 2003). SinceTable 6Concentration of trace metals in the tissue of four vegetables grown at di V erent rates of composted municipal waste (CMW) application Values in the same line for the same sample followed by the same letter are not signi W cantly di V erent as determined by Tukey’s test (P <0.05).aDry matter basis.bCoe Y cient of variation from the analysis of variance.mg/kg ¡1a CMW rate (t ha ¡1)CV b (%)0.012.525.050.0100.0Carrot (root)Fe 269.05a 219.38a 257.35a 259.95a 251.65a 32Mn 14.13a 16.01a 14.01a 11.67a 14.08a 36Cu 7.50a 6.96a 8.21a 7.42a 8.81a 19Cr0.37a 0.31a 0.38a 0.38a 0.39a 20Cauli X ower (X ower)Fe 101.80a 81.20ab 58.55bc 47.40c 53.23bc 26Mn 14.23a 14.50a 12.50a 12.22a 11.52a 11Cu 2.77a 2.25b 2.02b 2.08b 2.08b 10Co0.63a 0.63a 0.62a 0.68a 0.64a 17Sweet corn (seed)Fe 30.12a 26.34a 29.78a 29.32a 28.72a 31Mn 7.38a 8.96a 6.62a 6.36a 8.70a 22Cu 1.75a 1.93a 1.60a 1.78a 1.45a 14Cr 0.12a 0.14a 0.12a 0.14a 0.15a 26Ni 0.59a 0.37a 0.52a 0.57a 0.49a 75Pb0.37a 0.92a 0.50a 0.55a 0.81a 70Radish (root)Fe 2776.25a 2334.96a 3070.42a 2105.42a 3583.75a 47Mn 30.06a 28.94a 38.04a 24.72a 42.91a 39Cu 3.14b 5.20b 6.68b 5.53b 14.58a 50Ni 3.55a 2.11a 2.41a 2.25a 4.33a 57Co 3.40a 1.65a 2.41a 2.14a 2.84a 51Pb1.14b1.65ab3.39ab2.48ab7.24a79530 D.V. Pérez et al. / Bioresource Technology 98 (2007) 525–533most of the above processes occur in the upper layer of soil,only the results for the 0–5cm layer will be discussed here.It has been shown that contrary to the determination of the total content of any heavy metal in soil, chemical frac-tionation may provide more useful information concerning the mobility and bioavailability of heavy metals in soil (Tack and Verloo, 1995; Quevauviller, 1998). Sequential extraction procedures allow this fractionation by assuming that di V er-ent extracting solutions, applied in a speci W c sequence, will act on di V erent species or binding forms in the soil (Verloo and Eeckhout, 1990; López-Sánchez et al., 2002). The bind-ing form in the solid phase of the soil is related to the inten-sity of metal release to the liquid phase and hence likelihood of mobilization and bioavailability (Tack and Verloo, 1995).In the fractionation scheme used in this work (Table 2),fractions 1–3 are more likely to represent the equilibrium between the liquid and solid phases than fractions 4 and 5which are presumed to be the more structurally bound ele-ments occluded in Fe and Mn oxides, strongly adsorbed to Al oxides or residual forms of silicates.The concentrations of Fe, Mn, Cu, Cd, Ni, and Pb in the W ve fractions resultant from all treatments are presented in Fig.2. E xcept for Cu, most of the extracted metals were associated with the residual fraction (F5). The distribution of Cu was di V erent from that of other heavy metals. The organically bound fraction (F3) concentrated most of theCu analyzed in all treatments and the amount of Cu in F3has increased signi W cantly with increasing rates of CMW application. Although the other fractions were extracted in lower proportions, a signi W cant increase in the carbonate/exchangeable and the Al oxide +strong Fe/Mn oxide-bound fractions (F1 and F4, respectively) was observed towards the higher CMW applications. As it can be seen in Fig.2, despite the large amount of the residual fraction,Mn, Pb, and Cd also showed a signi W cant increase in the carbonate/exchangeable fraction (F1) with increasing CMW rates. The organically bound fraction (F3) has sig-ni W cantly increased for Pb and Cd as well.3.3.3. Humic fractionsRototilling the soil with no amendment application (0t ha ¡1) changed the humus composition signi W cantly com-pared to the soil before the experiment started (Table 8). The non-humic fraction (NHC) decreased, because the minerali-zation of the organic matter increased. The lowering of the total organic carbon (TOC) content corroborates this obser-vation (Table 8). The concentration of fulvic acids (FA)slightly increased as a consequence of this process of miner-alization. With the application of higher rates of CMW, the NHC content decreased, and, in the case of the 50t ha ¡1application, it was almost not detected. These results corrob-orate the W ndings of Kononova (1984) that soil cultivationTable 7Trace metal concentration of the soil one year after the growth of the four vegetables amended with composted municipal waste (CMW)Values in the same line for the same sample followed by the same letter are not signi W cantly di V erent as determined by Tukey’s test (P <0.05).aCoe Y cient of variation from the analysis of variance.CMW rate (t ha ¡1)Fe (£103) (mg kg ¡1)Mn (mg kg ¡1)Cu (mg kg ¡1)Cd (mg kg ¡1)Co (mg kg ¡1)Cr (mg kg ¡1)Ni (mg kg ¡1)Pb (mg kg ¡1)0–5cm 0.032.6290.711.4d 1.917.2ab 15.7b 17.519.2c 12.536.9314.220.7c 2.418.5a 21.6ab 21.226.9c 25.032.7299.724.6c 1.916.2ab 20.9ab 18.628.5c 50.035.6343.942.1b 2.416.5ab 27.1a 22.146.7b 100.033.9298.860.9a 2.512.5b 29.1a 21.260.2a CV (%)a129182131614145–10cm 0.035.2297.215.7c 1.816.5ab 17.717.423.0b 12.536.2300.017.6bc 2.416.8ab 19.419.924.9b 25.033.1317.416.0c 1.817.2a 18.318.029.8ab 50.036.4328.533.2ab 2.416.5ab 29.521.437.8ab 100.033.1290.546.4a 2.213.1b 26.018.147.4a CV (%)101128231123232510–20cm0.034.5313.216.0b 1.817.119.419.425.6ab 12.537.8304.116.7b 2.517.019.218.622.7b 25.028.6278.513.1b 2.415.816.018.118.9b 50.036.0326.027.5ab 2.218.322.619.533.5ab 100.032.9292.235.0a 2.116.121.918.137.6a CV (%)141630201720202420–40cm0.030.4308.210.6 1.617.415.3ab 18.2a 14.912.533.127712.1 2.416.015.8ab 15.4ab 15.925.032.2318.111.0 1.817.215.7ab 15.9ab 20.050.030.4273.09.9 1.816.613.3b 12.0b 15.5100.031.4278.914.8 1.816.819.1a 15.1b 20.2CV (%)14827228141132D.V. Pérez et al. / Bioresource Technology 98 (2007) 525–533531decreased the non-humic content of soil organic matter. In that case a slight increase of the humic acid fraction (HA)occurred, and, as a consequence, the fulvic acid content diminished. However, the most important e V ect observed was the increase of the humin fraction over the sum of the humic and fulvic acid fractions. This pattern was reportedTable 8Humic fractions extracted from the soil one year after the growth of the four vegetables amended with composted municipal waste (CMW)In parenthesis, results in percentage of the total organic carbon (TOC).aBefore cultivation, at the start of the experiment.CMW (t ha ¡1)Organic C in the humic fractionsTOC (g kg ¡1)FA free (g kg ¡1)FA bound (g kg ¡1)FA total (g kg ¡1)HA (g kg ¡1)Humin (g kg ¡1)FA +HA +humin (g kg ¡1)0.025.00.20 (8.0)0.19 (7.6)0.39 (15.6)0.12 (4.8) 1.78 (71.2) 2.29 (91.6)12.529.50.21 (7.1)0.20 (6.8)0.41 (13.9)0.15 (5.1) 2.12 (71.9) 2.68 (90.8)25.026.40.22 (8.3)0.10 (3.8)0.32 (12.1)0.16 (6.1) 1.93 (73.1) 2.41 (91.3)50.032.10.21 (6.5)0.15 (4.7)0.36 (11.2)0.21 (6.5) 2.63 (81.9) 3.20 (99.7)100.036.70.22 (6.0)0.16 (4.4)0.38 (10.4)0.23 (6.3) 2.92 (79.6) 3.53 (96.2)Control a35.20.28 (8.0)0.15 (4.3)0.43 (12.2)0.23 (6.5)1.80 (51.1)2.46 (69.9)。
宿州市耕地土壤养分时空变化特征分析
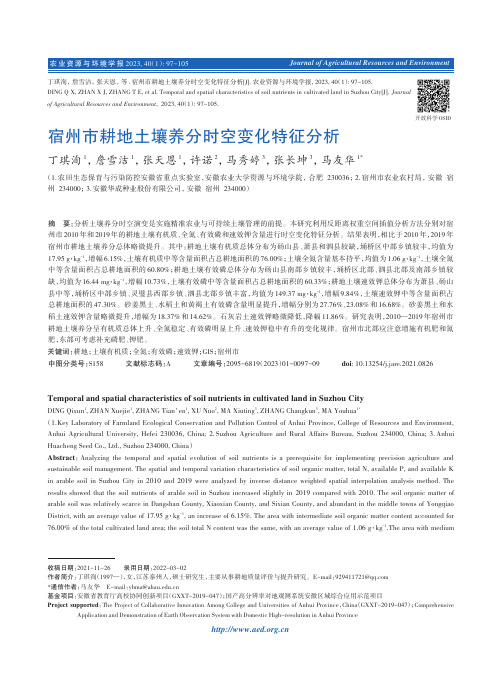
Temporal and spatial characteristics of soil nutrients in cultivated land in Suzhou CityDING Qixun 1,ZHAN Xuejie 1,ZHANG Tian′en 1,XU Nuo 2,MA Xiuting 3,ZHANG Changkun 3,MA Youhua 1*(1.Key Laboratory of Farmland Ecological Conservation and Pollution Control of Anhui Province,College of Resources and Environment,Anhui Agricultural University,Hefei 230036,China;2.Suzhou Agriculture and Rural Affairs Bureau,Suzhou 234000,China;3.Anhui Huacheng Seed Co.,Ltd.,Suzhou 234000,China )Abstract :Analyzing the temporal and spatial evolution of soil nutrients is a prerequisite for implementing precision agriculture and sustainable soil management.The spatial and temporal variation characteristics of soil organic matter,total N,available P,and available K in arable soil in Suzhou City in 2010and 2019were analyzed by inverse distance weighted spatial interpolation analysis method.The results showed that the soil nutrients of arable soil in Suzhou increased slightly in 2019compared with 2010.The soil organic matter of arable soil was relatively scarce in Dangshan County,Xiaoxian County,and Sixian County,and abundant in the middle towns of YongqiaoDistrict,with an average value of 17.95g·kg -1,an increase of 6.15%.The area with intermediate soil organic matter content accounted for76.00%of the total cultivated land area;the soil total N content was the same,with an average value of 1.06g·kg -1.The area with medium宿州市耕地土壤养分时空变化特征分析丁琪洵1,詹雪洁1,张天恩1,许诺2,马秀婷3,张长坤3,马友华1*(1.农田生态保育与污染防控安徽省重点实验室,安徽农业大学资源与环境学院,合肥230036;2.宿州市农业农村局,安徽宿州234000;3.安徽华成种业股份有限公司,安徽宿州234000)收稿日期:2021-11-26录用日期:2022-03-02作者简介:丁琪洵(1997—),女,江苏泰州人,硕士研究生,主要从事耕地质量评价与提升研究。
广东鹤山火烧迹地植被恢复后土壤养分含量变化
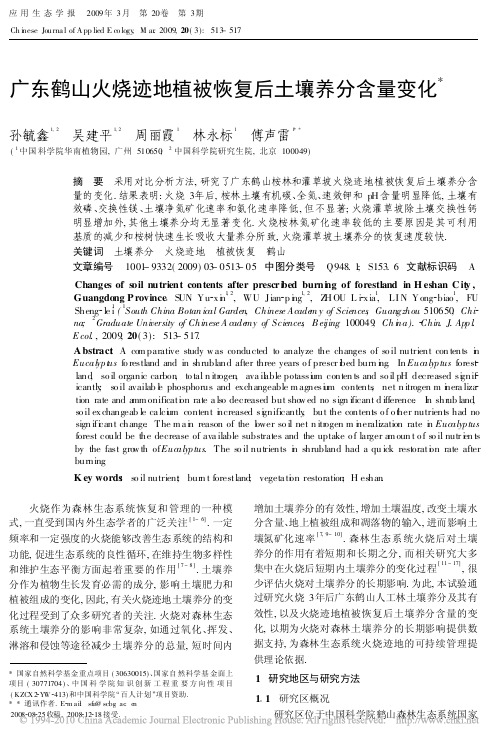
广东鹤山火烧迹地植被恢复后土壤养分含量变化*孙毓鑫1,2吴建平1,2周丽霞1林永标1傅声雷1**(1中国科学院华南植物园,广州510650;2中国科学院研究生院,北京100049)摘 要 采用对比分析方法,研究了广东鹤山桉林和灌草坡火烧迹地植被恢复后土壤养分含量的变化.结果表明:火烧3年后,桉林土壤有机碳、全氮、速效钾和pH 含量明显降低,土壤有效磷、交换性镁、土壤净氮矿化速率和氨化速率降低,但不显著;火烧灌草坡除土壤交换性钙明显增加外,其他土壤养分均无显著变化.火烧桉林氮矿化速率较低的主要原因是其可利用基质的减少和桉树快速生长吸收大量养分所致,火烧灌草坡土壤养分的恢复速度较快.关键词 土壤养分 火烧迹地 植被恢复 鹤山文章编号 1001-9332(2009)03-0513-05 中图分类号 Q948.1;S153.6 文献标识码 A Changes of soil nutrient contents after prescr i b ed burni n g of forestland in H eshan C it y ,Guangdong P rovince SUN Yu x i n 1,2,WU Jian p i n g 1,2,Z H OU L i x ia 1,LI N Yong biao 1,FUSheng le i 1(1South China Botan ical Garden,Chinese Acade m y of Sciences ,Guangzhou 510650,China;2Graduate University of Chinese A cade my of Sciences ,B eijing 100049,Ch i n a). Chin.J.App l .E col .,2009,20(3):513-517.A bstract :A co m parative study w as conducted to analyze the changes of so il nutrient con tents i n Eucal y p t u s fo restland and in shrubland after three years o f prescr i b ed burn i n g .In Eucalyp tus forest land ,so il organic carbon ,to tal n itr ogen ,ava il a ble potassi u m conten ts and so il pH decreased si g nif icantly ;so il availab l e phosphorus and exchangeable m agnesi u m contents ,net n itrogen m i n era liza tion rate and a mm onificati o n rate a lso decreased but sho w ed no sign ificant d ifference .I n shr ub land ,so il ex changeab le ca lci u m content i n creased si g nificantly ,but the contents o f o t h er nutrients had no sign ifi c ant change .The m a i n reason of the l o wer so il net n itr ogen m i n eralization rate i n Eucalyp tus forest could be the decrease of ava ilable substrates and the uptake o f larger a m oun t o f so il nutr i e n ts by the fast gro w th of Eucalyp tus .The so il nutrients i n shrubland had a qu ick restorati o n rate after burning .K ey words :so il nutrien;t bur n t forestland ;vegeta ti o n restoration ;H eshan .*国家自然科学基金重点项目(30630015)、国家自然科学基金面上项目(30771704)、中国科学院知识创新工程重要方向性项目(KZCX2 Y W 413)和中国科学院 百人计划 项目资助.**通讯作者.E m ai:l sf u@scbg .ac .c n 2008 08 25收稿,2008 12 18接受.火烧作为森林生态系统恢复和管理的一种模式,一直受到国内外生态学者的广泛关注[1-6].一定频率和一定强度的火烧能够改善生态系统的结构和功能,促进生态系统的良性循环,在维持生物多样性和维护生态平衡方面起着重要的作用[7-8].土壤养分作为植物生长发育必需的成分,影响土壤肥力和植被组成的变化,因此,有关火烧迹地土壤养分的变化过程受到了众多研究者的关注.火烧对森林生态系统土壤养分的影响非常复杂,如通过氧化、挥发、淋溶和侵蚀等途径减少土壤养分的总量,短时间内增加土壤养分的有效性,增加土壤温度,改变土壤水分含量、地上植被组成和凋落物的输入,进而影响土壤氮矿化速率[7,9-10].森林生态系统火烧后对土壤养分的作用有着短期和长期之分,而相关研究大多集中在火烧后短期内土壤养分的变化过程[11-17],很少评估火烧对土壤养分的长期影响.为此,本试验通过研究火烧3年后广东鹤山人工林土壤养分及其有效性,以及火烧迹地植被恢复后土壤养分含量的变化,以期为火烧对森林土壤养分的长期影响提供数据支持,为森林生态系统火烧迹地的可持续管理提供理论依据.1 研究地区与研究方法1 1 研究区概况研究区位于中国科学院鹤山森林生态系统国家应用生态学报 2009年3月 第20卷 第3期 Ch i nese Journa l of A pp lied E co logy ,M ar .2009,20(3):513-517野外科学观测研究站(简称鹤山站)内.该站位于广东省鹤山市中部(22!41∀N,112!54∀E ),平均海拔80m.试验样地处于鹤山站的共和试验区,占地面积约45hm 2,其中设有14个森林生态系统类型,每种类型均设3个重复,每块样地面积约1h m 2.该地区属南亚热带季风气候,年均气温21 7#,年均降水量1700mm ,年均蒸发量1600mm,年平均∃10#有效积温为7597#.地带性土壤为赤红壤,成土母质是砂页岩,土壤质地以粘壤土类和壤质粘粒为主,属强酸性土壤,pH 值在4 2~4 8.1 2 研究方法共和试验样地于2005年3月进行火烧处理.火烧前样地为荒草坡,火烧后人工种植尾叶桉(Euca l y p t u s urophy lla )、厚荚相思(Acacia crassicar pa )、阴香(C innam o m um bur m annii)和观光木(Tsoongio d en odorum )等树种.本试验选取4个不同处理:火烧桉林、未火烧桉林(对照C K )、火烧灌草坡和未火烧灌草坡(CK).每个类型试验区设有3个重复,共12个试验样地,每个样地面积约1h m 2.火烧桉林灌草丛覆盖率较低,桉林下灌丛和草坡的主要植被类型为芒萁(D icranop teris dichoto m a ).土壤氮矿化采用原状土就地连续培养法测定[18].试验周期为2008年3%5月,培养时间为30d .在每个样地的上、中、下3个坡度各选取地势平坦且具有代表性的3个点进行试验(n =9),每个点打入一组PVC 管,每组3支管,PVC 管长15c m 、直径5c m.其中一支立即取走带回实验室分析,得到当时的土壤氮状况,其余2支进行原位培养,一支管加盖,以避免雨淋,另一支管敞开,与自然条件变化一致.培养时间结束后,打入第2次的3支PVC 管,同时取走第1次打入的2支PVC 管和第2次打入的一支管,带回室内分析,其余2支留下进行培养.如此重复下去.各个坡位的3点混合成一个样品,挑出土壤样品中的植物根系和砂石,过2mm 筛,混合均匀,使土壤条件一致.称取10g 土样置于浸提瓶中,加入50m l2m o l &L -1KC l 溶液震荡1h ,过滤,提取液用流动注射分析仪(QC8000,美国LAC HAT 公司)分析土壤中NH 4+N 和NO 3- N 含量,土壤水分含量在105#下烘24h 测定,剩余样品在自然条件下风干,以供p H 、有机碳、全氮、全磷、有效磷、速效钾、交换性钙和镁的测定.土壤p H 值用土水比1∋2 5提取,p H 计测定;有机碳采用重铬酸钾氧化 外加热法;全氮经浓硫酸消煮后用流动注射仪分析;全磷用硫酸 高氯酸消解 钼锑抗比色法测定;有效磷用氟化铵 盐酸 钼锑抗比色法测定;速效K 和交换性Ca 2+、M g 2+用乙酸铵浸提后,分别用火焰光度法和原子吸收分光光度法测定[19].1 3 数据处理净氮矿化速率[m g &kg -1&(30d)-1]=培养30d 后的矿质氮(NH 4+ N +NO 3- N )-培养前的矿质氮(NH 4+N +NO 3- N )净硝化速率[m g &kg -1&(30d)-1]=培养30d 后的NO 3- N -培养前的NO 3- N净氨化速率[m g &kg -1&(30d)-1]=培养30d 后的NH 4+ N -培养前的NH 4+N数据测定结果均采用平均值,统计分析采用SPSS 11 5和Ex ce l 2003软件处理.显著性水平以P <0 05表示,采用单因素方差分析火烧对不同植被类型下土壤养分的影响.2 结果与分析2 1 火烧迹地土壤p H 的变化由图1可以看出,鹤山人工林土壤pH 值都小于4 3,属于强酸性土壤.与对照相比,火烧3年后,桉林和灌草坡的土壤pH 都有不同程度的下降,火烧桉林pH 值显著降低(P =0 024),火烧灌草坡p H 值也呈降低的趋势(P =0 1).2 2 火烧迹地土壤有机碳和全氮含量变化由表1可以看出,与对照样地相比,火烧3年后,桉林土壤有机碳含量显著降低(P =0 03),0~10c m 层土壤有机碳含量降低了38 6%;而灌草坡火烧迹地土壤有机碳含量没有显著变化.土壤全氮含量与土壤有机碳含量变化相似.与对照样地相比,火烧3年后,桉林土壤全氮含量减少了27 8%(P =图1 不同火烧迹地土壤p H 值变化Fig .1 Change o f so il p H i n diff e rent burn t sites .A :桉林Eu c a l yp t u s f orest ;B:灌草坡Sh rub l and .(:未火烧处理Un bu rnt ;):火烧处理Burn t .图中不同小写字母表示差异达0 05显著水平D i ff eren t lettersm eant si gn i fi cant d ifference at0 05leve.l 下同The sa m e bel o w.514应 用 生 态 学 报 20卷表1 不同火烧迹地的土壤有机碳和全氮含量Tab .1 Change of soil organ ic carbon and n itrogen in d if ferent burn t site s (m ean ∗SE ,n =9)火烧迹地Bur n t forestl and 处 理T reat m e nt 土壤有机碳S OC (g &kg -1)全氮TN (g &kg -1)碳氮比C /N桉林(23 54∗3 19a 0 72∗0 06a 31 60∗1 80Eucal yp t us f orest )14 45∗2 12b 0 52∗0 04b 26 54∗2 51灌草坡(18 97∗2 090 59∗0 0331 62∗2 39Shrubla nd)18 11∗2 350 62∗0 0528 36∗1 85(:未火烧处理Unburnt ;):火烧处理Bu rnt .同列数据不同小写字母表示差异达0 05显著水平D ifferen t l etters in t h e sa m e coloumn m ean t si gnifi cant differen t e at 0 05l eve.l0 015);而灌草坡火烧迹地土壤全氮没有显著变化.火烧迹地土壤碳氮比与对照相比都有不同程度的降低,但变化不明显.由图2可以看出,与对照样地相比,火烧桉林净氮矿化速率(NH 4+ N +NO 3- N )和氨化速率有降低的趋势,分别为0 3和0 54m g &kg -1&(30d)-1,但差异不显著(P =0 175和P =0 09);灌草坡火烧迹地0~10c m 土层净氮矿化速率、氨化速率和硝化速率之间差异不显著.火烧桉林净氮矿化速率较低图2 不同火烧迹地土壤氨化、硝化和净氮矿化速率的变化F i g .2 Chang e o f so il a mmon ificati on ,n itrificati on ,ne tN m i n e ra liza ti on ra te i n diff e rent burn t sites.5153期 孙毓鑫等:广东鹤山火烧迹地植被恢复后土壤养分含量变化的主要原因可能是土壤中可利用基质减少[20],以及桉树生长过程中快速消耗养分所致.2 3 火烧迹地土壤磷的变化由图3可以看出,火烧迹地土壤全磷和有效磷含量都有所降低.灌草坡火烧迹地与未火烧地土壤全磷之间差异接近于显著水平(P=0 072),桉林火烧迹地有效磷也接近于显著水平(P=0 078).土壤中磷被活化释放的最佳p H值为6 6~7 0[21],可能是火烧3年后,土壤p H值的降低影响了土壤中有效磷的释放.2 4 火烧迹地土壤阳离子的变化由图4可以看出,与对照样地相比,火烧桉林土壤速效钾含量从36 24m g&kg-1减少到23 22mg& kg-1,降低了35 93%(P=0 007);土壤交换性镁也接近显著降低(P=0 052),而交换性钙无显著差异.火烧灌草坡土壤交换性钙比对照增加了66%(P= 0 004),而土壤速效钾和交换性镁则无显著差异.3 讨论与结论土壤养分不仅影响植物群落的生长、发育和演替的速度,而且对生态系统的结构和功能有着重要影响.火烧通过挥发、氧化和淋溶等途径使土壤养分总量减少,短时间内增加了土壤养分的有效性[9].本研究中,火烧迹地土壤p H值低于未火烧地,其主要原因可能是:火烧后土壤水、热、气等微气候条件改变,光照增强,加速了表层腐殖质的分解,产生的矿质元素离子与土壤胶体表面吸附的氢离子发生交换后进入土壤溶液中,从而使土壤p H值降低[22-23].火烧3年后,桉林土壤有机碳和全氮含量都显著减少,与M onleon等[20]的研究结果相似.Guo 等[24]研究发现,火烧5年后0~10c m层土壤有机碳和全氮显著减少.Gundale等[25]研究发现,火烧3年后0~10c m层土壤碳和氮含量减少,但差异不显著.火烧对土壤碳和氮的影响取决于火烧强度和火烧时间[20,26],而气候、植被、地形等决定火烧迹地土壤碳和氮的恢复速度[8].火烧后桉林地上植被数量减少,凋落物的输入和根的周转率降低,从而使土壤碳和氮含量下降.此外,桉林的生长速率加快[27],从土壤中吸收了大量养分,也可能是导致土壤养分急剧下降的一个重要原因.NH4+ N和NO3- N是植物从土壤中吸收N的主要形态,研究火烧对土壤氮矿化作用的影响至关重要.火烧桉林净氮矿化作用呈现降低趋势,而火烧草坡几乎没有变化,因此火烧后可供桉林利用的有效氮减少.与Ada m s等[28]的研究相比,本研究中桉林的净氮矿化速率相当低,火烧后桉林净氮矿化作用进一步降低,将会影响桉林的生产力.P是南方酸性土壤中树木生长的重要限制因子之一,火烧迹地中P含量的变化对植物的生长发育有着重要作用[29].由于酸性土壤中易形成不溶于水的羟基磷酸盐,土壤有效磷含量将大大减少,随着土壤温度升高和土壤p H值降低,此过程会更加明显[9].土壤磷活化释放的最佳p H值在6 6~ 7 0[21],而火烧3年后土壤pH值的降低使得土壤中有效磷含量降低.K在土壤中流动性比较强,土壤速效钾含量水平是决定钾肥肥效的重要指标之一[25,30].火烧桉林土壤速效钾含量显著降低,可能是桉林生长吸收及淋溶流失所致.而Ca在土壤中流动性相对较小[25].火烧灌草坡交换性钙含量显著增加,火烧过程中Ca 转移到矿质层,随着时间的推移,灌草坡地上植被恢复,水土流失降低,使Ca在土壤中得到进一步积累.参考文献[1] Feeney SR,K o l b TE,Cov ing t on WW,et al.In fluenceo f th i nn i ng and burn i ng restoration treat m ents on presettl em ent pondero sa p i nes a t t he G us P earson N at ura lA rea.Canadian J ournal of Forest R esearch,1998,28:1295-1306[2] Hubbard RM,V ose J M,C li nton BD,et al.Stand restora ti on burn i ng i n oak p i ne forests in the sout hern A ppalach i ans:E ffects on aboveground b i om ass and carbonand n i trogen cyc li ng.F orest E cology and M anage m ent,2004,190:311-321[3] K aye JP,H art SC,Fu l e PZ,et al.Initial carbon,nitrogen,and phosphorus fl uxes fo llo w i ng ponderosa p i nerestoration treat m ents.E co l ogical A pp lica tions,2005,15:1581-1593[4] Boerner REJ,M orr i s S J,Sut her l and E K,et al.Spa ti a lva riab ilit y i n so il n itrogen dyna m i cs a fter prescri bedburning in Oh i o m i x ed oak fo nd scap e E cology,2000,15:425-439[5] D eng X B(邓晓保),Zou S Q(邹寿青),Fu X H(付先惠),et al.T he i m pacts o f l and use practices onthe co mmun iti es o f so il fauna i n the X ishuangbanna rai nforest,Y unnan,China.A cta E co l ogica S inica(生态学报),2003,23(1):130-138(i n Chi nese)516应 用 生 态 学 报 20卷[6] Scho ch P,B i nkley D.P rescr i bed burn i ng i ncreased nitrogen ava ilab ilit y in a m a t ure lobloll y p i ne stand.F orestE cology and M anag e m ent,1986,14:13-22[7] K ennard DK,G ho lzHL.Effects o f h i gh and lo w i ntensity fires on so il prope rti es and p l ant g row th i n a Bo li v iandry forest.P lant and Soil,2001,234:119-129[8] Certini G.Effects o f fire on properti es of forest so ils:Arev i ew.O ecolog ia,2005,143:1-10[9] Ca rterM C,Foster CD.P rescri bed burn i ng and productiv it y i n sout hern p i ne forests:A rev ie w.Forest Ecologyand M anage m ent,2004,191:93-109[10] W ilson C A,M itche ll R J,Bo ri ng LR,et al.So il n itrogen dyna m ics i n a fire m a i nta i ned forest ecosy stem:R esu lts over a3 yea r burn i nterva.l So il B iology&B ioche m is try,2002,34:679-689[11] Scheuner ET,M akeschin F,W e lls ED,et al.Sho rtte r m i m pacts o f harvesti ng and burni ng dist urbances onphysica l and chem ical character i sti cs o f f o rest so ils i nwestern N e w f oundland,Canada.Europ ean Journal ofF orest R esearch,2004,123:321-330[12] Abdel m enam M,W erner H,B etti na J,et al.E ffects o fprescri bed burn i ng on plant ava ilable nu trien ts i n dryhea t h l and ecosyste m s.P lant Ecology,2007,189:279-289[13] M oghaddas EEY,S tephens SL.T h i nn i ng,burn i ng,andth i n burn fuel treat m ent e ffects on so il properties in a S ierra N evada m i xed conife r f o rest.Forest Ecology andM anage m ent,2007,14:13-22[14] Cov i ngtonWW,Sacke tt SS.So ilm i neral nitrogen changes foll ow i ng prescribed burni ng i n ponde rosa pine.Forest E cology and M anage m ent,1992,54:175-191 [15] D a iW(戴 伟).Study on the changes i n so m e che m ica l so il properties a fter burn i ng.J ournal of B eij i ng Forestry U ni ver sity(北京林业大学学报),1994,16(1):102-105(i n Ch i nese)[16] Sha L Q(沙丽清),D eng J W(邓继武),X i e K J(谢克金),et al.Study on the change o f so il nutr i entbe fore and after burn i ng secondary forest i n X is huangbanna.A ct a P hy toeco logica S inica(植物生态学报),1998,22(6):513-517(i n Ch i nese)[17] W ang L(王 丽),K azuto S.R esea rch on t he dynam icchange o f so il nu trien ts i n the burned area o f m ounta i nf o res.t Bulleti n of Soil and W ater Conserva tion(水土保持通报),2008,28(1):81-85(in Ch i nese)[18] R a ison R J,ConnellM J,K hanna PK.M ethodology forst udy ing fl uxes of so ilm i neral N i n situ.So il B iology&B ioche m istry,1987,19:521-530[19] L i u G S(刘光崧),Jiang N H(蒋能慧),Zhang L D(张连第),et al.A na l ysis o f Soil Physical and Che m ica l P roperti es and D escri p tion of So il P ro fil es.Be iji ngChi na Standards Press,1996(i n Ch i nese)[20]M onleon V J,Cro m ack K,Landsberg JD.Sho rt andlong ter m effects o f prescr i bed unde rburn i ng on n i trogenava ilability in pondero sa p i ne stands i n centra l O regon.Canadian Journal of Forest R esearch,1997,27:367-378[21] G iard i na CP,Sanford RL,Do ckers m ith IC.Changes inso il phosphorus and n i trogen du ri ng slash and burnclearing o f a dry tropical forest.Soil Science Societ y ofAm er ican J ournal,2000,64:399-405[22] N eary DG,K l opatek CC,D eBano LF,et al.F ire effectson be lo w ground susta i nab ility:A rev ie w and synthesis.Forest Ecology and M anage m ent,1999,122:51-71 [23] L iu J X(刘菊秀),Zhou G Y(周国逸),Chu G W(褚国伟),et al.Effects of so il ac i d it y on the so il nutr i ents under D i nghushan monsoon ev eng reen broadleaved f o rest.A ct a Pedologica S i n ica(土壤学报),2003,40(5):763-767(i n Chinese)[24] G uo J F,Y ang Y S,Chen GS,et al.So ilC and N poolsin Chi nese fir and everg reen broad l ea f forests and t he irchanges w it h slash bu rning i n m i d subtrop i ca l Chi na.Pedosphere,2006,16:56-63[25] G undaleM J,D eluca TH,F ied l er CE,et al.R est o ra ti ontreat ments i n a M ontana pondero sa pi ne f o rest:Effectson so il physica,l che m ical and b i o l og ical properties.Forest Ecology and M anage m ent,2005,213:25-38 [26] Johnson DW,Curtis PS.E ffects of f o rest m anag e m enton so ilC and N st o rage:M eta ana l ys i s.Forest E co logyand M anage m en t,2001,140:227-238[27]G arc i a M onti e l DC,B ink l ey D.E ff ec t o f Eucalyp t ussali gna and A lbizia falcatar i a on so il pro cess and nitrogen supply i n H awa i.i O ecolog i a,1998,113:547-556 [28] A da m s MA,A tti w ill P M.N utr i ent cy cli ng and n i trogenm i nera li zati on i n euca lypt forests o f south eastern A ustralia:Indices o f n itrogen m i nera li za ti on.P l ant andSo il,1986,92:341-362[29] T ian K(田 昆).A study on the v ariati ons of phosphorus con tent i n fire slash.Journal of South w est Fores try College(西南林学院学报),1997,17(1):21-25(i n Chinese)[30] X u L F(许联芳),W ang K L(王克林),Zhu H H(朱捍华),et al.Effects o f d ifferen t l and use types onso il nutr i ents in karst reg i on of N orth w est Guangx.i Ch inese Journal of App lie d E co l ogy(应用生态学报),2008,19(5):1013-1018(in Chi nese)作者简介 孙毓鑫,男,1983年生,硕士研究生.主要从事恢复生态学和土壤生态学研究.E m ai:l sunyux i n@ 责任编辑 李凤琴5173期 孙毓鑫等:广东鹤山火烧迹地植被恢复后土壤养分含量变化。
血叶兰幼苗对干旱胁迫的生理响应及其抗旱性指标筛选
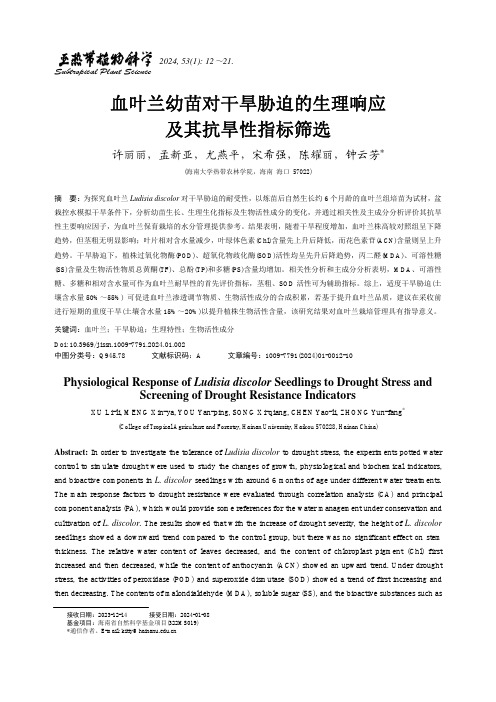
接收日期:2023-12-14 接受日期:2024-01-08基金项目:海南省自然科学基金项目(322MS019) *通信作者。
E-mail:*****************.cn血叶兰幼苗对干旱胁迫的生理响应及其抗旱性指标筛选许丽丽,孟新亚,尤燕平,宋希强,陈耀丽,钟云芳*(海南大学热带农林学院,海南 海口 57022)摘 要:为探究血叶兰Ludisia discolor 对干旱胁迫的耐受性,以炼苗后自然生长约6个月龄的血叶兰组培苗为试材,盆栽控水模拟干旱条件下,分析幼苗生长、生理生化指标及生物活性成分的变化,并通过相关性及主成分分析评价其抗旱性主要响应因子,为血叶兰保育栽培的水分管理提供参考。
结果表明,随着干旱程度增加,血叶兰株高较对照组呈下降趋势,但茎粗无明显影响;叶片相对含水量减少,叶绿体色素(Chl)含量先上升后降低,而花色素苷(ACN)含量则呈上升趋势。
干旱胁迫下,植株过氧化物酶(POD)、超氧化物歧化酶(SOD)活性均呈先升后降趋势,丙二醛(MDA)、可溶性糖(SS)含量及生物活性物质总黄酮(TF)、总酚(TP)和多糖(PS)含量均增加。
相关性分析和主成分分析表明,MDA 、可溶性糖、多糖和相对含水量可作为血叶兰耐旱性的首先评价指标,茎粗、SOD 活性可为辅助指标。
综上,适度干旱胁迫(土壤含水量50%~55%) 可促进血叶兰渗透调节物质、生物活性成分的合成积累,若基于提升血叶兰品质,建议在采收前进行短期的重度干旱(土壤含水量15%~20%)以提升植株生物活性含量,该研究结果对血叶兰栽培管理具有指导意义。
关键词:血叶兰;干旱胁迫;生理特性;生物活性成分Doi: 10.3969/j.issn.1009-7791.2024.01.002中图分类号:Q945.78 文献标识码:A 文章编号:1009-7791(2024)01-0012-10Physiological Response of Ludisia discolor Seedlings to Drought Stress andScreening of Drought Resistance IndicatorsXU Li-li, MENG Xin-ya, YOU Yan-ping, SONG Xi-qiang, CHEN Yao-li, ZHONG Yun-fang *(College of Tropical Agriculture and Forestry, Hainan University, Haikou 570228, Hainan China)Abstract: In order to investigate the tolerance of Ludisia discolor to drought stress, the experiments potted water control to simulate drought were used to study the changes of growth, physiological and biochemical indicators, and bioactive components in L. discolor seedlings with around 6 months of age under different water treatments. The main response factors to drought resistance were evaluated through correlation analysis (CA) and principal component analysis (PA), which would provide some references for the water management under conservation and cultivation of L. discolor . The results showed that with the increase of drought severity, the height of L. discolor seedlings showed a downward trend compared to the control group, but there was no significant effect on stem thickness. The relative water content of leaves decreased, and the content of chloroplast pigment (Chl) first increased and then decreased, while the content of anthocyanin (ACN) showed an upward trend. Under drought stress, the activities of peroxidase (POD) and superoxide dismutase (SOD) showed a trend of first increasing and then decreasing. The contents of malondialdehyde (MDA), soluble sugar (SS), and the bioactive substances such as2024, 53(1): 12~21.Subtropical Plant Science第1期许丽丽等:血叶兰幼苗对干旱胁迫的生理响应及其抗旱性指标筛选﹒13﹒total flavonoids (TF), total phenols (TP), and polysaccharides (PS) all increased. Through correlation analysis and principal component analysis, it was found that MDA, SS, PS, and relative water content could be used as the preferred evaluation indicators, and in addition, stem thickness and SOD activity could be used as auxiliary indicators for drought resistance evaluation in L. discolor. In summary, the results indicated that moderate drought stress (soil moisture content 50%–55%) could promote the synthesis and accumulation of osmotic regulating substances and bioactive components of L. discolor, At the same time, to improve the quality of L. discolor, it was recommended to carry out short-term severe drought (soil moisture content 15%–20%) before harvest so that the biological activities of L. discolor could be enhanced. These findings had practical guiding significance for cultivation management.Key words: Ludisia discolor; drought stress; physiological characteristics; biologically active ingredients干旱胁迫是影响植物生长发育和生理生化进程的关键性环境因素。
火山的爆发:地球的脉动 英语作文
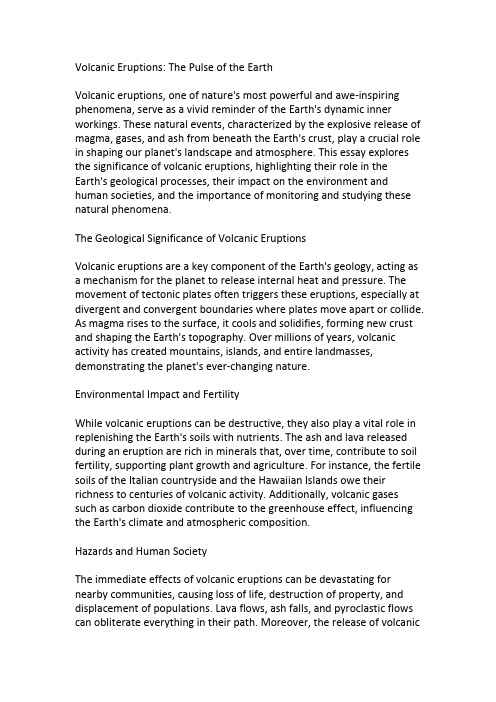
Volcanic Eruptions:The Pulse of the EarthVolcanic eruptions,one of nature's most powerful and awe-inspiring phenomena,serve as a vivid reminder of the Earth's dynamic inner workings.These natural events,characterized by the explosive release of magma,gases,and ash from beneath the Earth's crust,play a crucial role in shaping our planet's landscape and atmosphere.This essay explores the significance of volcanic eruptions,highlighting their role in the Earth's geological processes,their impact on the environment and human societies,and the importance of monitoring and studying these natural phenomena.The Geological Significance of Volcanic EruptionsVolcanic eruptions are a key component of the Earth's geology,acting as a mechanism for the planet to release internal heat and pressure.The movement of tectonic plates often triggers these eruptions,especially at divergent and convergent boundaries where plates move apart or collide. As magma rises to the surface,it cools and solidifies,forming new crust and shaping the Earth's topography.Over millions of years,volcanic activity has created mountains,islands,and entire landmasses, demonstrating the planet's ever-changing nature.Environmental Impact and FertilityWhile volcanic eruptions can be destructive,they also play a vital role in replenishing the Earth's soils with nutrients.The ash and lava released during an eruption are rich in minerals that,over time,contribute to soil fertility,supporting plant growth and agriculture.For instance,the fertile soils of the Italian countryside and the Hawaiian Islands owe their richness to centuries of volcanic activity.Additionally,volcanic gases such as carbon dioxide contribute to the greenhouse effect,influencing the Earth's climate and atmospheric composition.Hazards and Human SocietyThe immediate effects of volcanic eruptions can be devastating for nearby communities,causing loss of life,destruction of property,and displacement of va flows,ash falls,and pyroclastic flows can obliterate everything in their path.Moreover,the release of volcanicash into the atmosphere can lead to respiratory health issues,disrupt air travel,and affect climate patterns globally.The eruption of Mount Tambora in1815,for example,led to the"Year Without a Summer," causing widespread crop failures and famine across the globe. Monitoring and ResearchGiven the potential hazards associated with volcanic eruptions, monitoring and research are crucial for predicting and mitigating their impact.Volcanologists use a variety of tools,including seismographs,gas sensors,and satellite imagery,to monitor volcanic activity and provide early warnings to at-risk communities.Understanding the signs of an impending eruption,such as increased seismic activity or changes in gas emissions,can save lives and minimize economic damage. ConclusionVolcanic eruptions are a testament to the Earth's vitality,reminding us of the planet's constant evolution and the forces that shape our natural world.While they pose significant risks to human society,they also enrich our environment,contributing to the cycle of renewal that sustains life on Earth.By studying and monitoring volcanic activity,we can better appreciate the complexity of our planet and learn to coexist with these powerful natural phenomena.In embracing the pulse of the Earth,we acknowledge our place within a much larger,ever-changing system.。
红壤侵蚀地马尾松人工林恢复过程中土壤非保护性有机碳的变化_吕茂奎
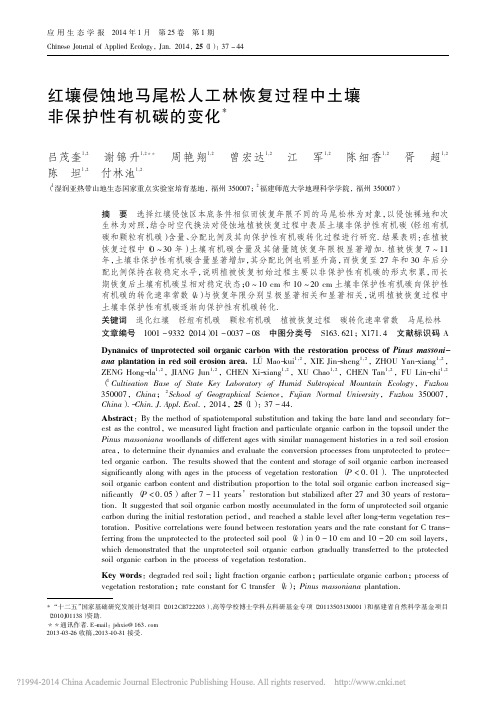
应用生态学报2014年1月第25卷第1期Chinese Journal of Applied Ecology,Jan.2014,25(1):37-44红壤侵蚀地马尾松人工林恢复过程中土壤非保护性有机碳的变化*吕茂奎1,2谢锦升1,2**周艳翔1,2曾宏达1,2江军1,2陈细香1,2胥超1,2陈坦1,2付林池1,2(1湿润亚热带山地生态国家重点实验室培育基地,福州350007;2福建师范大学地理科学学院,福州350007)摘要选择红壤侵蚀区本底条件相似而恢复年限不同的马尾松林为对象,以侵蚀裸地和次生林为对照,结合时空代换法对侵蚀地植被恢复过程中表层土壤非保护性有机碳(轻组有机碳和颗粒有机碳)含量、分配比例及其向保护性有机碳转化过程进行研究.结果表明:在植被恢复过程中(0 30年)土壤有机碳含量及其储量随恢复年限极显著增加.植被恢复7 11年,土壤非保护性有机碳含量显著增加,其分配比例也明显升高,而恢复至27年和30年后分配比例保持在较稳定水平,说明植被恢复初始过程主要以非保护性有机碳的形式积累,而长期恢复后土壤有机碳呈相对稳定状态;0 10cm和10 20cm土壤非保护性有机碳向保护性有机碳的转化速率常数(k)与恢复年限分别呈极显著相关和显著相关,说明植被恢复过程中土壤非保护性有机碳逐渐向保护性有机碳转化.关键词退化红壤轻组有机碳颗粒有机碳植被恢复过程碳转化速率常数马尾松林文章编号1001-9332(2014)01-0037-08中图分类号S163.621;X171.4文献标识码ADynamics of unprotected soil organic carbon with the restoration process of Pinus massoni-ana plantation in red soil erosion area.L Mao-kui1,2,XIE Jin-sheng1,2,ZHOU Yan-xiang1,2,ZENG Hong-da1,2,JIANG Jun1,2,CHEN Xi-xiang1,2,XU Chao1,2,CHEN Tan1,2,FU Lin-chi1,2(1Cultivation Base of State Key Laboratory of Humid Subtropical Mountain Ecology,Fuzhou350007,China;2School of Geographical Science,Fujian Normal University,Fuzhou350007,China).-Chin.J.Appl.Ecol.,2014,25(1):37-44.Abstract:By the method of spatiotemporal substitution and taking the bare land and secondary for-est as the control,we measured light fraction and particulate organic carbon in the topsoil under thePinus massoniana woodlands of different ages with similar management histories in a red soil erosionarea,to determine their dynamics and evaluate the conversion processes from unprotected to protec-ted organic carbon.The results showed that the content and storage of soil organic carbon increasedsignificantly along with ages in the process of vegetation restoration(P<0.01).The unprotectedsoil organic carbon content and distribution proportion to the total soil organic carbon increased sig-nificantly(P<0.05)after7-11years’restoration but stabilized after27and30years of restora-tion.It suggested that soil organic carbon mostly accumulated in the form of unprotected soil organiccarbon during the initial restoration period,and reached a stable level after long-term vegetation res-toration.Positive correlations were found between restoration years and the rate constant for C trans-ferring from the unprotected to the protected soil pool(k)in0-10cm and10-20cm soil layers,which demonstrated that the unprotected soil organic carbon gradually transferred to the protectedsoil organic carbon in the process of vegetation restoration.Key words:degraded red soil;light fraction organic carbon;particulate organic carbon;process ofvegetation restoration;rate constant for C transfer(k);Pinus massoniana plantation.*“十二五”国家基础研究发展计划项目(2012CB722203)、高等学校博士学科点科研基金专项(20113503130001)和福建省自然科学基金项目(2010J01138)资助.**通讯作者.E-mail:jshxie@163.com2013-03-26收稿,2013-10-31接受.在全球气候变化背景下,如何恢复退化土壤的固碳能力是当今生态学和土壤学关注的焦点问题和优先研究领域之一.造林和改善农林业的管理是增加退化土壤碳固定和减缓大气CO2浓度增加的重要方法[1],具有成本低、潜力大和可持续性等优点.目前我国土壤退化严重,增加退化土壤的碳汇已成为我国亟待解决的重大问题.我国南方红壤区是仅次于黄土高原的第二大侵蚀退化区,尤其是花岗岩发育的红壤大面积严重退化,碳密度低,固碳潜力大[2],增加侵蚀退化红壤的固碳能力对于我国气候变化国际谈判具有重要意义.福建省长汀县是我国南方典型的红壤侵蚀区.20世纪80年代至今,在当地政府的重视下,该区先后对该退化生态系统进行了大量的恢复与重建工作,昔日的“红色沙漠”如今已是满山翠绿,极大地改善了当地的生态环境[2].但是,有关植被恢复过程中土壤有机碳动态变化以及土壤有机碳固定的长期有效性等问题尚不清楚.因此,研究生态恢复过程中侵蚀地土壤有机碳库动态及调控过程对后续的生态恢复与保护具有重要价值.土壤有机碳(soil organic carbon,SOC)是表征土壤质量和健康状况的重要指标[3].但是在侵蚀地植被恢复过程中,土壤总有机碳积累的短期变化并不能指示生态恢复对土壤碳动态的影响.因此,识别更敏感的活性有机碳组分有助于阐明土壤有机碳的动态.土壤非保护性有机碳(unprotected SOC)是活性有机碳的一部分,由新近凋落的、部分分解的、与土壤矿质结合不紧的植物残体组成,主要包括轻组有机碳(light fraction organic carbon,LFOC)和颗粒有机碳(particulate organic carbon,POC)[4].非保护性有机碳对土地利用或植被覆盖变化的响应非常敏感,可以作为土壤有机碳库变化的灵敏指标[5-8].鉴于此,本研究应用土壤有机碳物理分组方法,结合时空代换法,探讨红壤侵蚀地不同恢复年限马尾松人工林表层土壤的轻组有机碳和颗粒有机碳含量及其分配比例的变化,以及土壤碳库的演变过程,以期为今后侵蚀地的生态恢复及重建提供科学依据.1研究地区与研究方法1.1试验地概况长汀县河田镇地处福建省西南部、汀江上游(25ʎ33'—25ʎ48'N,l16ʎ18'—116ʎ31'E),海拔300 500m.该地区属中亚热带季风气候,年均气温17.5 19.2ħ,年均降雨量1700mm,年均蒸发量1403mm,年均无霜期260d,年均日照时数1924.6h,≥10ħ积温4100 4650ħ.该地区地形为开阔的河谷盆地,四周被低山高丘所环抱;土壤主要为中粗粒花岗岩发育的红壤,可蚀性较高,原有地带性植被(常绿阔叶林)基本破坏殆尽,现有植被以马尾松(Pinus massoniana)次生林和人工林为主.花岗岩风化壳深厚,一般为10m,最深处达百米,降雨侵蚀严重,加之植被的大面积破坏,使得河田镇成为全国水土流失最严重的地区之一,许多地方的表层土壤已被剥蚀殆尽,后期植被恢复极为困难.本研究选取土壤母岩和成土条件相同、地形及地表生态过程相似的6个不同恢复年限的试验样地,其中7a与8a、10a与11a治理样地分别作为治理7a和10a的重复.以侵蚀裸地(CK1)和次生林(CK2)为恢复前、后的对照.样地治理前的土壤侵蚀状况以及土壤有机质含量与侵蚀裸地基本一致.因此,不同恢复年限马尾松林土壤有机质的差异主要由植被恢复和治理措施引起的植物生长条件及土壤生态过程的差异造成.试验地类型及基本概况为:1)侵蚀裸地(CK1):海拔315m,坡度为12ʎ,坡向NE35ʎ.土壤侵蚀度一般达强度以上,表层土壤流失殆尽,B层出露,表层土壤(0 20cm)有机质含量为1.4 2.5g·kg-1;地表植被以稀疏的马尾松小老头树(平均胸径4.3 6.6cm,平均树高3.1 4.6m)以及少量芒萁(Dicranopteris dichotoma)和野古草(Arundinclla setosa)为主;2)伯湖和乌石岽:海拔分别为318和378m,坡度18ʎ和22ʎ,坡向ES30ʎ和SW20ʎ,分别于2003和2004年开始治理,通过7 8年的封禁治理,林下植被覆盖度接近100%,马尾松平均胸径和树高分别为8.2cm和7.3m;3)石官凹和游坊:海拔分别为314和328m,坡度15ʎ和21ʎ,坡向SW20ʎ和ES25ʎ,分别于2000和2001年对原有低效马尾松林进行小水平沟整地,补植胡枝子(Lespedeza bicolor),后期封禁管护.经过10 11年的封禁治理,林下植被盖度分别达到85%和70%以上,马尾松平均胸径分别为10.4和7.5cm,树高分别为7.5和5.9m;4)水东坊:海拔310m,坡度18ʎ,坡向NE50ʎ,于1984年对其水平沟整地,补植黑荆,林下套种胡枝子,并进行封禁管护,林下植被覆盖度97%,马尾松平均胸径和树高分别为16.9cm和14.3m;5)八十里河:海拔310m,坡度18ʎ,坡向NE50ʎ,于1981年对其进行小水平沟整地,保留原有的马尾松等乔木,并在马尾松林下套种胡枝子、紫穗槐(Amorpha fruticosa).经过30年的人工恢复,林83应用生态学报25卷下植被覆盖度97%,马尾松平均胸径和树高分别为16.9cm和14.3m;6)次生林(CK2):海拔321m,坡度10ʎ,坡向WN10ʎ,主要树种为马尾松、木荷(Schi-ma superba)针阔混交林,林龄约70 110年(异林龄),林下植被覆盖度95%以上,马尾松平均胸径和树高分别为47.5cm和19.0m.1.2研究方法1.2.1土样采集2011年7月在每块试验地设3个20mˑ20m标准地,分别进行本底和生物量调查.在每个标准地上用内径5cm土钻按“S”型随机、等量、多点混合取样,取8 10个点,每个样地取3组0 10和10 20cm土层混合土样,带回室内,过2mm筛、风干后备用.土壤容重用环刀法测定.1.2.2土壤有机碳含量测定SOC含量采用浓硫酸-重铬酸钾高温外加热氧化法测定,土壤全氮采用全自动凯氏定氮法测定,轻组和颗粒有机碳氮采用碳氮元素分析仪(Elementar VarioEL III,德国)测定,SOC储量采用土壤容重和有机碳含量推算[9].1.2.3轻组有机碳的分离及分配比例参照Janzen 等[10]的分离方法,称取风干土样10g,放在100mL 离心管中,加入50mL NaI(1.7g·cm-3)重液,震荡30min后离心,然后用真空管吸取悬浮部分,通过微孔滤膜过滤,继续往离心管中加20 30mL NaI,重复上述过程2 3次,直至没有可见的轻组物质,至少用75mL0.01mol·L-1CaCl2冲洗以除去轻组中NaI,另加100 150mL去离子水冲洗轻组.然后将滤纸上的轻组洗到预先称量的器皿中,在60ħ下烘干,称量,计算烘干样品占总土壤样品质量的比例,再取出部分样品用于分析SOC含量.根据轻组质量和有机碳含量,计算LFOC数量.以LFOC数量除以SOC总量,得到LFOC的分配比例[11].1.2.4颗粒有机碳分离及分配比例称取风干土样20g,放入250mL塑料瓶中,加入100mL浓度为5g·L-1的六偏磷酸钠[(NaPO3)6]溶液,手摇15min,再用震荡器震荡18h(90r·min-1).将土壤悬液过53μm筛,用蒸馏水反复冲洗后,所有留在筛子上的物质在60ħ下烘干至恒量,计算该部分占整个土壤样品质量的比例.通过分析烘干样品中SOC,结合>53μm的颗粒质量计算POC数量.以POC数量除以SOC总量得到POC的分配比例[11].1.3数据处理土壤非保护性有机碳向保护性有机碳转化的速率常数(k)用下式计算:k=P/(TTp U)式中:U为非保护性有机碳碳库(颗粒与轻组有机碳的均值);P为保护性有机碳库(总有机碳与非保护性有机碳的差值);TTp为保护性有机碳周转时间,设为100年[11].所有数据处理和统计分析均在Excel2003和SPSS17.0软件下进行,方差分析采用LSD法,采用一元线性回归模型建立两个变量之间的相关关系,显著性水平设定为α=0.05.用Origin7.5和Excel2003软件作图.2结果与分析2.1植被恢复过程中土壤有机碳含量及其储量的变化由图1可以看出,马尾松人工林恢复过程中,侵蚀地表层(0 20cm)SOC含量和储量总体呈非线性增长,其中以0 10cm土层的增长更显著.在植被恢复7 11年时,与侵蚀裸地(CK1)相比,010cm SOC含量及其储量均极显著增加,而10 20cm SOC含量及其储量差异不显著.但在植被恢复7 11年间,0 10和10 20cm SOC含量及其储量随年限增加并无递增的趋势,且不同恢复年限之间无显著差异,可能与不同恢复年限侵蚀地的治理措图1植被恢复过程中土壤有机碳含量及储量Fig.1Storage and content of the soil organic carbon in theprocess of vegetation restoration(meanʃSD).CK1:侵蚀裸地Erosive bare land;CK2:次生林Secondary forest.下同The same below.不同小写字母表示同一土层不同恢复年限林地间的差异显著(P<0.05)Different letters in the same soil layer denoted sig-nificant difference between the woodlands with different restoration yearsat0.05level.931期吕茂奎等:红壤侵蚀地马尾松人工林恢复过程中土壤非保护性有机碳的变化施不同以及试验地间的差异有关;在植被恢复27年和30年后,0 10cm SOC含量及其储量均显著高于CK1及恢复7 11年的样地;而10 20cm土层SOC含量及其储量显著高于CK1,但与恢复7 11年的样地无显著差异.这说明植被恢复优先影响0 10cm土层,随着治理年限的增加,下层土壤SOC 进一步积累.在植被恢复30年后,SOC含量及其储量仍显著低于恢复后的次生林(CK2),说明侵蚀地仍有较大的固碳潜力.2.2植被恢复过程中土壤轻组有机碳及其分配的变化植被恢复过程中LFOC并未随恢复年限呈线性上升(图2).轻组含量变化幅度较大,尤其是恢复7 11年,0 10和10 20cm土层轻组含量从CK1(1.2 2.2g·kg-1)增加到3.4 10.6g·kg-1(表1),0 10cm土层LFOC含量也极显著增加,是CK1(0.69g·kg-1)的4.5 4.7倍;而10 20cm土层与CK1无显著差异.在植被恢复27和30年后,其LFOC含量与7 11年无显著差异,但在这一阶段LFOC含量随恢复年限呈上升趋势,且逐渐趋近于CK2.植被恢复过程中,LFOC占总有机碳的比例也呈现不同程度的变化.总体上,恢复7 11年土壤轻组有机碳的分配比例(LFOC/SOC)明显高于治理27年后(图2),0 10cm土层LFOC/SOC从CK1(29.1%)增加到36.6% 41.9%,且0 10和10 20cm土层的变化趋势一致.当植被恢复7 11年,LFOC很大程度上决定了土壤总有机碳含量,且以0 10cm最为明显,其决定了总有机碳的25.9% 49.0%.植被恢复27和30年后LFOC/SOC逐渐保持在一定范围内(17.2% 21.6%),说明随着恢复年限增加,土壤有机碳组分逐渐趋于稳定.2.3植被恢复过程中土壤颗粒有机碳及其分配的变化植被恢复过程中土壤POC与LFOC的变化趋势相同(图2),且POC含量略高于LFOC含量.在植被恢复7 10年时,0 10cm土层颗粒物含量大幅度增加,与CK1(3.7g·kg-1)相比,增加了4.4 8.3倍,与恢复27和30年样地间无显著差异(表1);然而,在整个植被恢复过程中,10 20cm土层颗粒物含量的变化相对较小,不同恢复年限的样地间均无显著差异.土壤POC含量也呈相同的变化趋势,恢复前11年土壤POC大量积累,从0.22 g·kg-1增至2.99g·kg-1;而恢复至27年后,POC 的积累速率相对减小.在植被恢复27和30年后,0 10和10 20cm土层POC含量均呈增加趋势,且0 10cm土层增加较显著,比CK1(0.75g·kg-1)增加了4.8倍以上.植被恢复过程中,土壤POC分配比例(POC/ SOC)呈现不同程度的变化.总体上,恢复7 11年图2植被恢复过程中土壤轻组和颗粒有机碳含量及其占总有机碳的比例Fig.2Contents of LFOC,POC and their proportions to total soil organic carbon in the process of vegetation restoration(meanʃSD).04应用生态学报25卷表1植被恢复过程中土壤轻组和颗粒有机质组分含量及其碳(C)含量Table1Carbon contents of soil light matter and particulate organic matter in the process of the vegetation restoration (g·kg-1)组分Fraction 土层Soillayer(cm)侵蚀裸地Erosive bare land(CK1)OM C恢复年限Restoration years7OM C10OM C27OM C30OM C次生林Secondary forest(CK2)OM CLFOM0 102.2318.39.9325.510.6301.97.4300.211.7276.614.2272.710 201.2292.73.4322.03.4304.01.8301.53.0265.77.1265.6 POM0 103.7200.619.4169.630.6135.227.2133.326.4185.533.0203.310 201.4160.59.1130.922.1125.216.595.315.2106.517.0133.0 LFOM:轻组有机质Soil light fraction matter;POM:颗粒有机质Particulate organic matter.OM:有机物质Organic matter.表2土壤总有机碳和非保护性有机碳与恢复年限及其相互之间的相关方程Table2Correlation equations between the total SOC,unprotect SOC and restoration years土壤有机碳SOC(g·kg-1)0 10cm10 20cm轻组有机碳LFOC(g·kg-1)0 10cm10 20cm颗粒有机碳POC(g·kg-1)0 10cm10 20cm年限Year(a)y=0.463x+3.340(r=0.972,P=0.016)y=0.144x+1.980(r=0.986,P=0.005)y=0.018x+2.277(r=0.670,P=0.225)y=0.011x+0.678(r=0.787,P=0.075)y=0.041x+2.377(r=0.929,P=0.027)y=0.017x+0.827(r=0.914,P=0.038)土壤有机碳SOC(g·kg-1)y=0.116x+1.499(r=0.791,P=0.071)y=0.126x+0.335(r=0.809,P=0.056)y=0.210x+0.946(r=0.993,P=0.000)y=0.223x+0.167(r=0.975,P=0.004)轻组有机碳LFOC(g·kg-1)y=0.725x+0.033(r=0.941,P=0.002)y=0.415x+0.104(r=0.861,P=0.022)图3土壤非保护性有机碳向保护性有机碳转化速率常数(k)与植被恢复年限的相关关系Fig.3Correlation between rate constant for C transfer from theunprotected to the protected soil pool(k)and restoration years.的变化幅度明显大于恢复27和30年后(图2),且两个土层变化趋势相似.植被恢复7 11年时,POC占据土壤有机碳的主要部分,0 10和10 20cm土层分别占总有机碳的38.3%和30.7%;随着植被恢复年限的增加,POC/SOC逐渐降低,恢复至27和30年后,两个土层POC/SOC分别减少至26.7%和29.5%,接近于CK2(25.3%和23.5%).这说明植被恢复27和30年后,土壤POC占SOC的比例逐渐趋于稳定.2.4恢复年限对土壤非保护性有机碳的影响相关分析表明,土壤轻组与颗粒有机碳含量在很大程度上取决于土壤总有机碳的含量(62.6%98.7%)(表2),且0 10cm土层POC含量与总有机碳含量呈极显著线性相关,说明LFOC和POC占据了总有机碳重要的部分;尤其是植被恢复7 11年,土壤总有机碳的13.2% 41.9%由非保护碳构成.两个土层有机碳含量与恢复年限呈显著或极显著线性相关.土壤非保护性有机碳含量与恢复年限之间呈线性相关(表2).其中POC含量与恢复年限之间达到显著相关水平.随恢复年限的增加,010cm土层非保护性有机碳向保护性有机碳的转化速率常数增加,而10 20cm土层达到显著相关水平(图3).这说明随着植被恢复年限增加,土壤中非保护性有机碳逐渐向保护性有机碳转化.3讨论3.1植被恢复对土壤有机碳及非保护性有机碳的影响本研究中,随着植被恢复年限的增加,SOC含量及其储量均呈增加趋势,且在植被恢复前11年,林地SOC含量及其储量发生不同程度的变化.这是因为植被恢复过程中,林地植被覆盖度明显增加,促进14 1期吕茂奎等:红壤侵蚀地马尾松人工林恢复过程中土壤非保护性有机碳的变化了马尾松生长.这一方面直接减少了SOC的流失,另一方面,通过凋落物及死亡根系的养分归还,增加了土壤有机物质的输入量[2,12].SOC积累的最大速率通常发生在土地利用方式或土地管理变化的初期[13],因此,在植被恢复初期SOC含量的变化较大.另外,生态恢复过程中土壤理化性质得到明显改善,微生物随恢复年限的增加发生显著变化,经过20 30年处于基本稳定[14].每年大量的枯枝落叶和营养元素等物质重新返回到生态系统中,且随着植被恢复演替的进行,有机物质输入量逐渐增多,为微生物提供了可利用的碳源、氮源,促进了微生物活性及微生物生物量的升高;另一方面,微生物数量的增加加速了枯落物及死细根的分解,促进养分归还,从而显著提高了SOC含量及其储量.LFOC对植被类型、土地利用方式、施肥及经营措施等非常敏感[15].本研究发现,植被恢复过程中土壤LFOC含量呈非线性增加趋势,可能与不同恢复年限样地治理措施及施肥制度的差异有关.其中0 10和10 20cm土层LFOC含量变化范围分别为0.69 3.24和0.35 1.13g·kg-1,均逐渐接近CK2,但相对低于川西典型植被类型(0.84 10.85 g·kg-1)[16]、暖温带次生林(0.86 15.21 g·kg-1)、落叶松人工林(0.54 14.17g·kg-1)[6]和亚热带格氏栲天然林(<9g·kg-1)[7].在植被恢复初期,施肥、整地等治理措施促进了马尾松和林下植被的快速生长[17],林地植被覆盖率明显提高,建立了凋落物和细根的物质循环途径,为轻组有机质提供了物质来源,因此土壤LFOC含量显著增加.此外,轻组有机碳占总有机碳29.1% 41.9%,接近落叶松人工林(29.3% 32.8%)[6],但高于暖温带次生林(19.0% 23.1%)[6]和川西典型植被类型(4.0% 13.4%)[16].植被恢复27年后LFOC分配比例开始下降,且保持在16.9%左右,接近于CK2(16.5%)和暖温带次生林(19.0% 23.1%)[6]的水平.这可能是植被恢复到一定程度后,土壤中物质来源与土壤微生物的分解保持在一个相对平稳的状态;植被恢复过程中土壤和轻组有机质C/N呈先增加后降低的趋势(图4),这反映了植被恢复过程中土壤有机碳的分解程度[9,18],同时,在分解过程中常发生N的微生物固定[19],因此土壤中轻组有机碳的积累速率明显高于总有机碳,引起植被恢复初期轻组有机碳分配比例较高及C/N比值升高.植被恢复过程中土壤POM与LFOM含量及其分配比例呈基本一致的趋势(图2).土壤POM含量(0.22 5.83g·kg-1)与山地常绿阔叶林和常绿落叶阔叶混交林(1.11 8.96g·kg-1)[16]、暖温带次生林(0.76 8.8g·kg-1)和落叶松人工林(0.89 10.64g·kg-1)[6]土壤颗粒有机碳含量相接近.颗粒有机碳占总有机碳的比例(17.0% 43.7%)接近于Degryze等[20](17% 38%)、Jr Garten[21](9% 27%)及Conant等[22](13% 31%)的研究结果.颗粒有机碳对气候条件、农业耕作与管理方式、土地利用类型变化及施肥等外界条件十分敏感[23],而且土壤有机质短期内的变化与波动主要发生在易氧化分解的那一部分[24-25],导致植被恢复初期土壤颗粒含量波动也较大.3.2土壤非保护性有机碳与植被恢复年限的耦合作用植被恢复过程中,土壤非保护性有机碳的积累速度明显高于土壤总有机碳,且土壤LFOM和POM 与土壤总有机碳呈线性正相关关系.土壤轻组和颗粒有机碳尽管是由不同测定方法获得,但两者之间呈显著线性相关关系(表2),表明它们在一定程度上能够指示土壤碳库及其稳定性的变化[6-8,26].因图4植被恢复过程中土壤、轻组和颗粒有机质C/N的变化Fig.4Changes of the C/N ratio of LFOM,POM and SOM in the process of vegetation restoration(meanʃSD).24应用生态学报25卷此,土壤非保护性有机碳可以反映植被恢复过程土壤有机碳的变化.植被恢复过程中土壤非保护性有机碳占总有机碳的比例先增加后趋于平缓,在恢复27年后土壤非保护性有机碳逐渐接近于CK2,说明土壤有机碳的输入与微生物分解过程逐渐达到相对平衡状态,植被恢复过程中土壤、轻组和颗粒有机质C/N的变化(图4)也可以证实这一点.因为高C/N的土壤有机质分解缓慢,微生物活性低,利于有机质的积累,而且土壤碳积累的最大速率通常发生在土地利用或土地管理变化的初期[13,18].另外,0 10和10 20cm 层土壤非保护性有机碳向保护性有机碳转化速率常数(k)与植被恢复年限显著相关(图3),即植被恢复过程中非保护性有机碳逐渐向保护性有机碳转化,说明在红壤侵蚀区,依靠人工生态修复和自肥作用来恢复土壤有机碳库质量,以及维持土壤有机碳的长期有效性是可能的.然而,土壤碳库需要漫长的时间才能达到平衡状态,因此仍需加强林地管理及合理疏伐来减少土壤水分胁迫,同时还应引入演替后续物种,促进植物群落的拓殖与更替[14].参考文献[1]IPCC.Land use,land-use change,and forestry//Wat-sonRT,Noble LR,Bolin B,eds.A SpecialReport ofthe Intergovernmental Panel on Climate Change.Cam-bridge:Cambridge University Press,2000[2]Xie JS,Guo JF,Yang ZJ,et al.Rapid accumulation of carbon on severely eroded red soils through afforestationin subtropical China.Forest Ecology and Management,2013,300:53-59[3]Ding G,Novak JM,Amarasiriwardena D,et al.Soil organic matter characteristics as affected by tillage man-agement.Soil Science Society of America Journal,2002,66:421-429[4]Six J,ConantRT,Paul EA,et al.Stabilization mecha-nisms of soil organic matter:Implications for C-satura-tion of soils.Plant and Soil,2002,241:155-176[5]Skjemstad JO,Taylor JA,Janik LJ,et al.Soil organic carbon dynamics under long-term sugarcane monocul-ture.Australian Journal of SoilResearch,1999,37:151-164[6]Wu J-G(吴建国),Zhang X-Q(张小全),Wang Y-H (王彦辉),et al.The effects of land use changes on thedistribution of soil organic carbon in physical fraction ofsoil.Scientia Silvae Sinicae(林业科学),2002,38(4):19-29(in Chinese)[7]Yang Y-S(杨玉盛),Liu Y-L(刘艳丽),Chen G-S(陈光水),et al.Content and distribution of unprotectedsoil organic carbon in natural and monoculture plantationforests of Castanopsis kawakamii in subtropical China.Acta Ecologica Sinica(生态学报),2004,24(1):1-8(in Chinese)[8]Jiang P-K(姜培坤).Soil active carbon pool under dif-ferent types of vegetation.Scientia Silvae Sinicae(林业科学),2005,41(1):10-13(in Chinese)[9]State Forestry Administration(国家林业局).Forest Soil Analysis Methods:Determination of Organic Matterin Forest Soil and Calculation of Carbon-NitrogenRatio(LY-T12371999).Beijing:China Standards Press,1999(in Chinese)[10]Janzen HH,Campbell CA,Brandt SA,et al.Light-fraction organic matter in soils from long-term crop rota-tions.Soil Science Society of America Journal,1992,56:1799-1806[11]Jr Garten CT,Post WM,Hanson PJ,et al.Forest soil carbon inventories and dynamics along an elevation gra-dient in the southern Appalachian Mountains.Biogeo-chemistry,1999,45:115-145[12]Li G-D(李国栋),Liu G-Q(刘国群),Zhuang S-Y (庄舜尧),et al.Changes of organic matter in soilsplanted Lei bamboo with different years.Chinese Journalof Soil Science(土壤通报),2010,41(4):245-249(in Chinese)[13]Smith P,Powlson DS.Glendining MJ,et al.Potential for carbon sequestration in European soils:Preliminaryestimates for five scenarios using results from long-termexperiments.Global Change Biology,1997,3:67-79[14]Xue S(薛?),Liu G-B(刘国彬),Dai Q-H(戴全厚),et al.Dynamic changes of soil microbial biomassin the restoration process of shrub plantations in loesshilly area.Chinese Journal of Applied Ecology(应用生态学报),2008,19(3):517-523(in Chinese)[15]Xie J-S(谢锦升),Yang Y-S(杨玉盛),Xie M-S(谢明曙),et al.Advance of research on light fraction or-ganic matter in soil.Journal of Fujian College of Forest-ry(福建林学院学报),2006,26(3):281-288(inChinese)[16]Xiang C-H(向成华),Luan J-W(栾军伟),Luo Z-S (骆宗诗),et al.Labile soil organic carbon distributionon influenced by vegetation types along an elevation gra-dient in west Sichuan,China.Acta Ecologica Sinica(生态学报),2010,30(4):1025-1034(in Chi-nese)[17]Xie J-S(谢锦升),Chen G-S(陈光水),He Z-M(何宗明),et al.Growth characteristics of Pinus massoni-ana under different improving patterns in degraded redsoil.Bulletin of Soil and Water Conservation(水土保持341期吕茂奎等:红壤侵蚀地马尾松人工林恢复过程中土壤非保护性有机碳的变化通报),2001,21(6):24-27(in Chinese)[18]Fissore C,Giardina CP,KolkaRK,et al.Temperature and vegetation effects on soil organic carbon qualityalong a forested mean annual temperature gradient inNorth America.Global Change Biology,2008,14:193-205[19]Recous S,Aita C,Mary B.In situ changes in gross N transformations in bare soil after addition of straw.SoilBiology and Biochemistry,1999,31:119-133[20]Degryze S,Six J,Paustian K,et al.Soil organic carbon pool changes following land-use conversions.GlobalChange Biology,2004,10:1120-1132[21]Jr Garten CT.Soil carbon storage beneath recently es-tablished tree plantation in Tennessee and South Caro-lina,USA.Biomass and Bioenergy,2002,23:93-102[22]ConantRT,Six J,Paustian K.Land use effects on soil carbon fractions in the southeastern United States.I.Management-intensive versus extensive grazing.Biologyand Fertility of Soils,2003,38:386-392[23]Xie J-S(谢锦升),Yang Y-S(杨玉盛),Chen G-S(陈光水),et al.Advances on soil particulate organicmatter.Journal of SubtropicalResources and Environ-ment(亚热带资源与环境学报),2009,4(4):43-52(in Chinese)[24]Biederbeck BO,ZentnerRP.Labile soil organic matter as influenced by cropping practices in an arid environ-ment.Soil Biology and Biochemistry,1994,26:1647-1656[25]Kalbitz K,Solinger S,Park JH,et al.Controls on the dynamics of dissolved organic matter in soils:A review.Soil Science,2000,165:277-304[26]Tang G-M(唐光木),Xu W-L(徐万里),Sheng J-D (盛建东),et al.The variation of soil organic carbonand soil particle-size in Xinjiang oasis farmland of differ-ent years.Acta Pedologica Sinica(土壤学报),2010,47(2):279-285(in Chinese)作者简介吕茂奎,男,1986年生,硕士研究生.主要从事森林水文与生态恢复研究.E-mail:228lmk@163.com责任编辑李凤琴44应用生态学报25卷。
覆土控鞭栽培对高节竹鞭根养分和抗性生理特征的影响
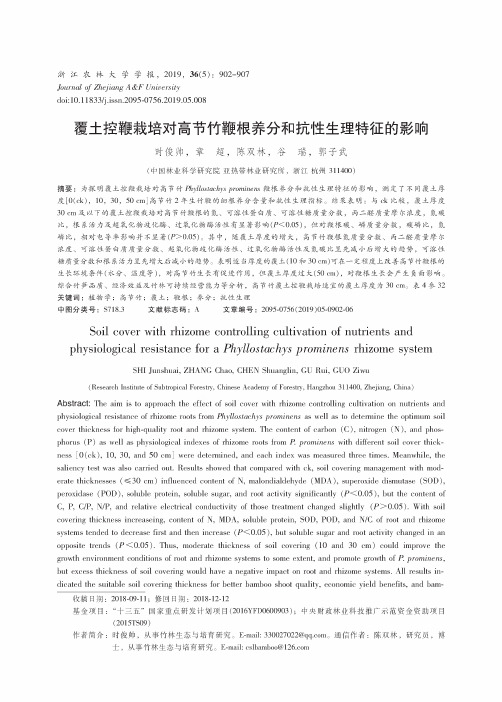
浙江农林大学学报,2019,36(5):902-907Journal qf Zhejiang A&1Universitydoi:10.11833/j.issn.2095-0756.2019.05.008覆土控鞭栽培对高节竹鞭根养分和抗性生理特征的影响时俊帅,章超,陈双林,谷瑞,郭子武(中国林业科学研究院亚热带林业研究所,浙江杭州311400)摘要:为探明覆土控鞭栽培对高节竹Phyllostachys prominens鞭根养分和抗性生理特征的影响,测定了不同覆土厚度[0(ck),10,30,50cm'高节竹2年生竹鞭的细根养分含量和抗性生理指标。
结果表明:与ck比较,覆土厚度30cm及以下的覆土控鞭栽培对高节竹鞭根的氮、可溶性蛋白质、可溶性糖质量分数,丙二醛质量摩尔浓度,氮碳比,根系活力及超氧化物歧化酶、过氧化物酶活性有显著影响(P<0.05),但对鞭根碳、磷质量分数,碳磷比,氮磷比,相对电导率影响并不显著(P>0.05)。
其中,随覆土厚度的增大,高节竹鞭根氮质量分数、丙二醛质量摩尔浓度、可溶性蛋白质质量分数、超氧化物歧化酶活性、过氧化物酶活性及氮碳比呈先减小后增大的趋势,可溶性糖质量分数和根系活力呈先增大后减小的趋势。
表明适当厚度的覆土(10和30cm)可在一定程度上改善高节竹鞭根的生长环境条件(水分、温度等),对高节竹生长有促进作用,但覆土厚度过大(50cm),对鞭根生长会产生负面影响(综合竹笋品质、经济效益及竹林可持续经营能力等分析,高节竹覆土控鞭栽培适宜的覆土厚度为30cm o表4参32关键词:植物学;高节竹;覆土;鞭根;养分;抗性生理中图分类号:S718.3文献标志码:A文章编号:2095-0756(2019)05-0902-06Soil cover with rhizome controlling cultivation of nutrients and physiological resistance for a Phyllostachys prominens rhizome systemSHI Junshuai,ZHANG Chao,CHEN Shuanglin,GU Rui,GUO Ziwu(Research Institute of Subtropical Forestry,Chinese Academy of Forestry,Hangzhou311400,Zhejiang,China)Abstract:The aim is to approach the effect of soil cover with rhizome controlling cultivation on nutrients and physiological resistance of rhizome roots from Phyllostachys prominens as well as to determine the optimum soil cover thickness for high-quality root and rhizome system.The content of carbon(C),nitrogen(N),and phosphorus(P)as well as physiological indexes of rhizome roots from P.prominens with different soil cover thickness[0(ck),10,30,and50cm]were determined,and each index was measured three times.Meanwhile,the saliency test was also carried out.Results showed that compared with ck,soil covering management with moderate thicknesses(W30cm)influenced content of N,malondialdehyde(MDA),superoxide dismutase(SOD), peroxidase(POD),soluble protein,soluble sugar,and root activity significantly(P V0.05),but the content of C,P,C/P,N/P,and relative electrical conductivity of those treatment changed slightly(P>0.05).With soil covering thickness increaseing,content of N,MDA,soluble protein,SOD,POD,and N/C of root and rhizome systems tended to decrease first and then increase(P V0.05),but soluble sugar and root activity changed in an opposite trends(P<0.05).Thus,moderate thickness of soil covering(10and30cm)could improve the growth environment conditions of root and rhizome systems to some extent,and promote growth of P.prominens, but excess thickness of soil covering would have a negative impact on root and rhizome systems.All results indicated the suitable soil covering thickness for better bamboo shoot quality,economic yield benefits,and bam-收稿日期:2018-09-11;修回日期:2018-12-12基金项目:“十三五+国家重点研发计划项目(2016YFD0600903);中央财政林业科技推广示范资金资助项目(2015TS09)作者简介:时俊帅,从事竹林生态与培育研究,E-mail:330027022@ o通信作者:陈双林,研究员,博士,从事竹林生态与培育研究,E-mail:cslbamboo@第36卷第5期时俊帅等:覆土控鞭栽培对高节竹鞭根养分和抗性生理特征的影响903boo forest sustainable management of P.prominens should be30cm.[Ch,4tab.32ref.]Key words:botany;Phyllostachys prominens;soil cover with rhizome controlling cultivation;root and rhizome system;nutrient;physiological system of resistance根系是植物吸收养分和水分的主要器官,对环境变化十分敏感,而土壤环境的变化会时刻影响到植物根系的生理过程$其中,土壤温度是决定植物细胞呼吸速率的重要影响因子,影响根系对水分和养分的吸收$2年生脂松Pinus resinosa在根际温度8!时产生新根的数量最少,16!时最多,新根总长度与温度在8~20!内呈正相关[1]$欧洲水青冈Fagus sylvaiica细根的呼吸作用对土壤温度的依赖性十分显著[2];土壤紧实度影响土壤的通气条件和水分状况,会对植物根系的生长发育产生影响$油松Pinus tabuliformis种子的发芽率和出苗率与土壤含水量密切相关[3]$林木根系周围的土壤相对含水率影响着地上、地下部分生物量。
Bioremediationof...

Bioremediation of Pb-contaminated soil by incubating with Phanerochaete 土壤重金属修复外文文献译文通过加入黄孢原毛平革菌和稻草培养的方式对铅污染土壤的生物修复实验室进行了利用培养好的白腐菌和秸秆对被铅污染的土壤进行生物修复模拟。
监测了土壤的pH值,铅浓度,土壤微生物,微生物代谢商,微生物商和微生物生物量C和N的比值。
以上指标用来学习土壤中铅的强度和微生物在生物修复过程中的影响。
研究表明被施以白腐菌和秸秆的土壤含有更低的可溶性交换铅,更低的生物商和生物量C和N的比值(0 mg /kg干土,1.9 mg CH2-C,生物量C 和4.9 在60天时),和更高的微生物生物量和微生物代谢商(2258 mg /kg 干土和7.86% 在第60天)。
另外,在logistic等式中的动力参数是用BIOLOG数据进行计算。
对动力参数进行分析后,就能得到一些微生物群的微生物量的信息。
所有数据显示含铅土壤的生物利用度被减少,这样潜在铅的强度被缓解,并且土壤微生物影响和微生物群的微生物量有所提高。
1. 简介土壤中的重金属是最常见的环境污染。
铅被认定是所有重金属中危害最为严重的。
铅污染的主要来源是采矿、冶炼、含铅汽油、污水污泥、废弃电池以及其他含铅产品。
这些种类繁多的铅来源导致土壤中含铅量偏高。
Linet al的报道指出在瑞典Falun西南部大量工厂废物聚集地,土壤含铅量超1000 mg /kg。
Buatier et al.指出在法国一个污染地,地表铅浓度达到460–2670 mg/kg。
铅的毒性和生物利用度受土壤pH、氧化还原和铅种类的影响。
土壤中的含铅化合物主要通过可交换物、碳酸盐类、Fe/Mn 氧化物有机物和残留态流失。
可溶性可交换状态铅的最大危害是铅非常容易浸入地下水,地表以及农作物。
然而铅在有机物和残留状态却无害,这是由于有机健的强度和硫化物,特别是在重污染土壤中。
植酸修饰复合生物炭对镉污染稻田土壤的钝化效果研究
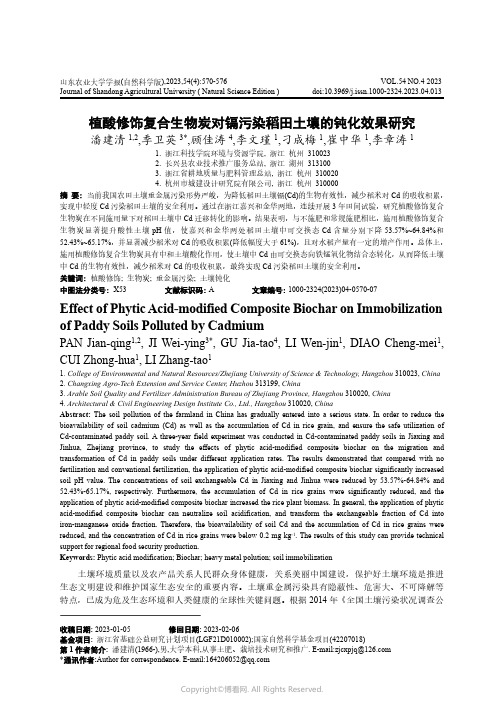
山东农业大学学报(自然科学版),2023,54(4):570-576VOL.54NO.42023 Journal of Shandong Agricultural University(Natural Science Edition)doi:10.3969/j.issn.1000-2324.2023.04.013植酸修饰复合生物炭对镉污染稻田土壤的钝化效果研究潘建清1,2,季卫英3*,顾佳涛4,李文瑾1,刁成梅1,崔中华1,李章涛11.浙江科技学院环境与资源学院,浙江杭州3100232.长兴县农业技术推广服务总站,浙江湖州3131003.浙江省耕地质量与肥料管理总站,浙江杭州3100204.杭州市城建设计研究院有限公司,浙江杭州310000摘要:当前我国农田土壤重金属污染形势严峻,为降低稻田土壤镉(Cd)的生物有效性,减少稻米对Cd的吸收积累,实现中轻度Cd污染稻田土壤的安全利用。
通过在浙江嘉兴和金华两地,连续开展3年田间试验,研究植酸修饰复合生物炭在不同施用量下对稻田土壤中Cd迁移转化的影响。
结果表明,与不施肥和常规施肥相比,施用植酸修饰复合生物炭显著提升酸性土壤pH值,使嘉兴和金华两处稻田土壤中可交换态Cd含量分别下降53.57%~64.84%和52.43%~65.17%,并显著减少稻米对Cd的吸收积累(降低幅度大于61%),且对水稻产量有一定的增产作用。
总体上,施用植酸修饰复合生物炭具有中和土壤酸化作用,使土壤中Cd由可交换态向铁锰氧化物结合态转化,从而降低土壤中Cd的生物有效性,减少稻米对Cd的吸收积累,最终实现Cd污染稻田土壤的安全利用。
关键词:植酸修饰;生物炭;重金属污染;土壤钝化中图法分类号:X53文献标识码:A文章编号:1000-2324(2023)04-0570-07Effect of Phytic Acid-modified Composite Biochar on Immobilization of Paddy Soils Polluted by CadmiumPAN Jian-qing1,2,JI Wei-ying3*,GU Jia-tao4,LI Wen-jin1,DIAO Cheng-mei1, CUI Zhong-hua1,LI Zhang-tao11.College of Environmental and Natural Resources/Zhejiang University of Science&Technology,Hangzhou310023,China2.Changxing Agro-Tech Extension and Service Center,Huzhou313199,China3.Arable Soil Quality and Fertilizer Administration Bureau of Zhejiang Province,Hangzhou310020,China4.Architectural&Civil Engineering Design Institute Co.,Ltd.,Hangzhou310020,ChinaAbstract:The soil pollution of the farmland in China has gradually entered into a serious state.In order to reduce the bioavailability of soil cadmium(Cd)as well as the accumulation of Cd in rice grain,and ensure the safe utilization of Cd-contaminated paddy soil.A three-year field experiment was conducted in Cd-contaminated paddy soils in Jiaxing and Jinhua,Zhejiang province,to study the effects of phytic acid-modified composite biochar on the migration and transformation of Cd in paddy soils under different application rates.The results demonstrated that compared with no fertilization and conventional fertilization,the application of phytic acid-modified composite biochar significantly increased soil pH value.The concentrations of soil exchangeable Cd in Jiaxing and Jinhua were reduced by53.57%-64.84%and 52.43%-65.17%,respectively.Furthermore,the accumulation of Cd in rice grains were significantly reduced,and the application of phytic acid-modified composite biochar increased the rice plant biomass.In general,the application of phytic acid-modified composite biochar can neutralize soil acidification,and transform the exchangeable fraction of Cd into iron-manganese oxide fraction.Therefore,the bioavailability of soil Cd and the accumulation of Cd in rice grains were reduced,and the concentration of Cd in rice grains were below0.2mg kg-1.The results of this study can provide technical support for regional food security production.Keywords:Phytic acid modification;Biochar;heavy metal polution;soil immobilization土壤环境质量以及农产品关系人民群众身体健康,关系美丽中国建设,保护好土壤环境是推进生态文明建设和维护国家生态安全的重要内容。
滇池流域软土灾害效应分析及应对措施
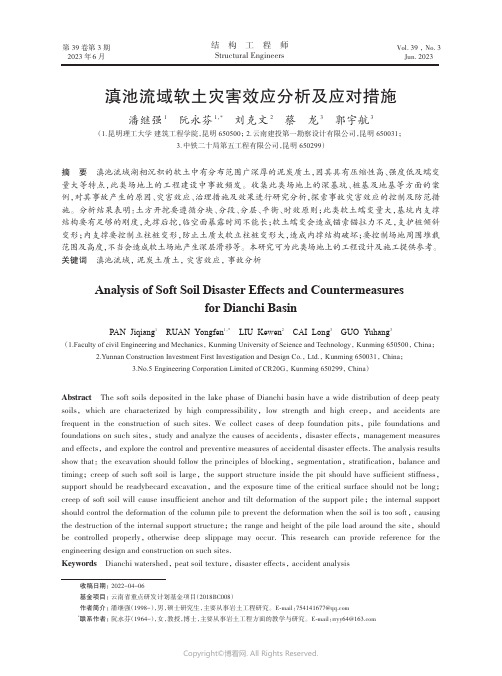
第 39 卷第 3 期2023 年6 月结构工程师Structural Engineers Vol. 39 , No. 3Jun. 2023滇池流域软土灾害效应分析及应对措施潘继强1阮永芬1,*刘克文2蔡龙3郭宇航3(1.昆明理工大学建筑工程学院,昆明 650500; 2.云南建投第一勘察设计有限公司,昆明 650031;3.中铁二十局第五工程有限公司,昆明 650299)摘要滇池流域湖相沉积的软土中有分布范围广深厚的泥炭质土,因其具有压缩性高、强度低及蠕变量大等特点,此类场地上的工程建设中事故频发。
收集此类场地上的深基坑、桩基及地基等方面的案例,对其事故产生的原因、灾害效应、治理措施及效果进行研究分析,探索事故灾害效应的控制及防范措施。
分析结果表明:土方开挖要遵循分块、分段、分层、平衡、时效原则;此类软土蠕变量大,基坑内支撑结构要有足够的刚度,先撑后挖,临空面暴露时间不能长;软土蠕变会造成锚索锚拉力不足,支护桩倾斜变形;内支撑要控制立柱桩变形,防止土质太软立柱桩变形大,造成内撑结构破坏;要控制场地周围堆载范围及高度,不当会造成软土场地产生深层滑移等。
本研究可为此类场地上的工程设计及施工提供参考。
关键词滇池流域,泥炭土质土,灾害效应,事故分析Analysis of Soft Soil Disaster Effects and Countermeasuresfor Dianchi BasinPAN Jiqiang1RUAN Yongfen1,*LIU Kewen2CAI Long3GUO Yuhang3(1.Faculty of civil Engineering and Mechanics, Kunming University of Science and Technology, Kunming 650500, China;2.Yunnan Construction Investment First Investigation and Design Co., Ltd., Kunming 650031, China;3.No.5 Engineering Corporation Limited of CR20G, Kunming 650299, China)Abstract The soft soils deposited in the lake phase of Dianchi basin have a wide distribution of deep peaty soils,which are characterized by high compressibility,low strength and high creep,and accidents are frequent in the construction of such sites. We collect cases of deep foundation pits,pile foundations and foundations on such sites, study and analyze the causes of accidents, disaster effects, management measures and effects, and explore the control and preventive measures of accidental disaster effects. The analysis results show that: the excavation should follow the principles of blocking, segmentation, stratification, balance and timing;creep of such soft soil is large,the support structure inside the pit should have sufficient stiffness,support should be readybecard excavation, and the exposure time of the critical surface should not be long;creep of soft soil will cause insufficient anchor and tilt deformation of the support pile;the internal support should control the deformation of the column pile to prevent the deformation when the soil is too soft, causing the destruction of the internal support structure; the range and height of the pile load around the site, should be controlled properly,otherwise deep slippage may occur. This research can provide reference for the engineering design and construction on such sites.Keywords Dianchi watershed, peat soil texture, disaster effects, accident analysis收稿日期:2022-04-06基金项目:云南省重点研发计划基金项目(2018BC008)作者简介:潘继强(1998-),男,硕士研究生,主要从事岩土工程研究。
微生物对红树林沉积物中As形态分布的效应及机制
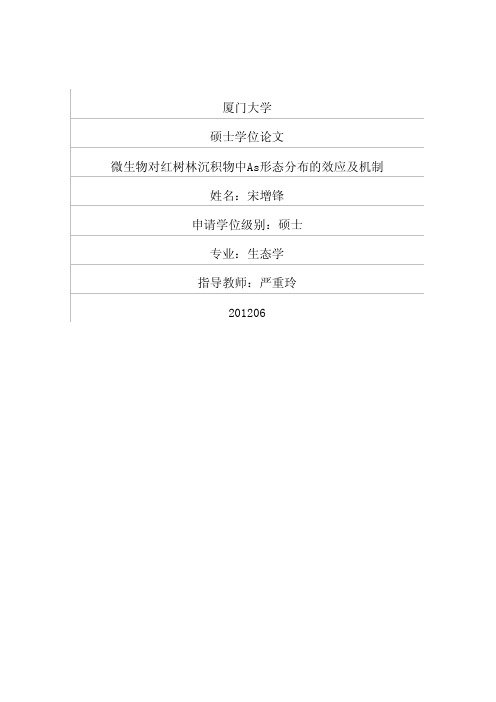
厦门大学硕士学位论文微生物对红树林沉积物中As形态分布的效应及机制姓名:***申请学位级别:硕士专业:生态学指导教师:***201206摘要摘要红树林沉积物是具有高还原性、高硫含量、高有机质、高粘粒含量等特点的独特生境,同时又是重金属污染物的重要源和汇。
As在生态系统中有很强的毒性,对动植物都有很大的潜在威胁。
沉积物中As吸附形态的分布直接影响到As污染物的生物毒性.。
当前对于As污染的研究多集中在陆地作物土壤;对于红树林沉积物中As形态分布的研究尚不多见。
有鉴于此,本研究以典型红树林沉积物为样本,在不同浓度As(0,10,30,60,90mg·kg。
)及沉积物微生物(1.甲醛灭菌:c组:2.施mini培养基:P组;3.无处理:n组。
)处理下,采用连续提取法提取并测定沉积物中As不同形态的含量。
同时分别检测微生物生物量,铁还原菌、硫化细菌、硫酸盐还原菌等功能菌的数量,土壤酶活性等指标。
以期探讨As在红树林沉积物中吸附形态的分布,并揭示沉积物中微生物对As形态分布的效应及可能机制;为红树林湿地As污染治理提供了理论依据和科学支撑。
本文主要研究结果如下:红树林沉积物中舢吸附形态的分布有其独特的特点:沉积物中残渣态As(F5)所占的比重是最多的,含量在50.O%以上;次之是铁锰氧化态As(F3)其含量从6.3%到46.9%,易受环境影响;再次是有机及硫化物结合态As(F4),其含量从4.O%到30.6%,易受微生物影响;而后则是碳酸盐结合态As(F2),含量可达16.2%7.含量最少的就是可交换态AS(F1)和水溶态As(F0),F1含量最高的不到8.3%(p组,0mg·kg-1处理下),而FO含量最少,含量仅为0.07%.1.38%。
随着As处理浓度的增加,As的形态分布也有所改变:水溶态(Fo)As百分含量随着处理浓度的增加而增大,从0.07%直增到1.38%;可交换态(F1)百分含量则随着处理浓度的增大而呈现下降趋势,(c、n、P三组变化趋势相似);而F2在c组中其百分含量是逐渐增大的,在n和P组则呈现出先升后降的趋势,高浓度的As对碳酸盐结合态(F2)的含量是有一定影响的;在c组中铁锰氧化态(F3)百分含量是先升后降,到30mg·kg"1时达到最大值,在n组和P组其含量大大减少,甚至达到70%左右;F4含量在c组中含量较为稳定,但在11组P组中其含量有显著的增加,尤其是在60.90mg·kg。
土附着在大地上 材料作文
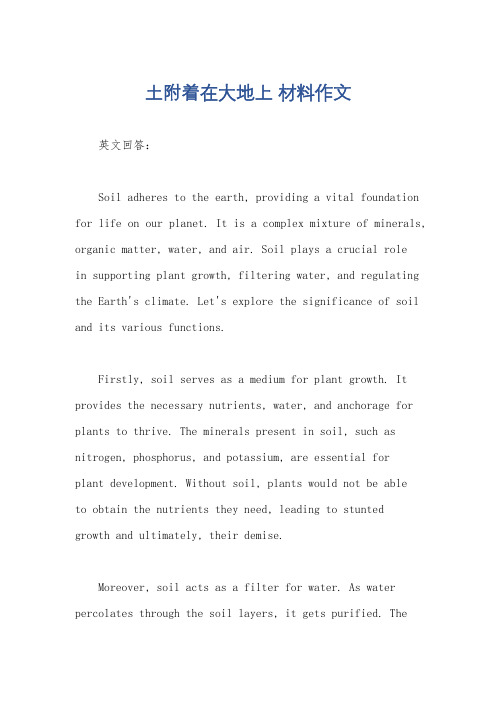
土附着在大地上材料作文英文回答:Soil adheres to the earth, providing a vital foundation for life on our planet. It is a complex mixture of minerals, organic matter, water, and air. Soil plays a crucial rolein supporting plant growth, filtering water, and regulating the Earth's climate. Let's explore the significance of soil and its various functions.Firstly, soil serves as a medium for plant growth. It provides the necessary nutrients, water, and anchorage for plants to thrive. The minerals present in soil, such as nitrogen, phosphorus, and potassium, are essential forplant development. Without soil, plants would not be ableto obtain the nutrients they need, leading to stuntedgrowth and ultimately, their demise.Moreover, soil acts as a filter for water. As water percolates through the soil layers, it gets purified. Thesoil particles and organic matter trap pollutants and contaminants, preventing them from reaching groundwater sources. This natural filtration process helps to maintain the quality of our drinking water and protect aquatic ecosystems.Additionally, soil plays a crucial role in regulating the Earth's climate. It acts as a carbon sink, absorbingand storing carbon dioxide from the atmosphere. This helpsto mitigate the effects of climate change by reducing the amount of greenhouse gases in the air. Soil also acts as a buffer against extreme temperatures, providing insulation and preventing heat loss from the Earth's surface.Furthermore, soil supports a diverse range of organisms. From earthworms to bacteria, countless microorganismsinhabit the soil and contribute to its fertility. These organisms break down organic matter, releasing nutrientsand improving soil structure. They also help to control pests and diseases, promoting healthy plant growth.In conclusion, soil is a precious resource that isessential for sustaining life on Earth. Its functions in supporting plant growth, filtering water, regulating the climate, and supporting biodiversity are vital for thewell-being of our planet. We must recognize the importanceof soil conservation and sustainable land management practices to ensure its preservation for future generations.中文回答:土壤附着在大地上,为地球上的生命提供了重要的基础。
花岗岩红壤团聚体稳定性对土地利用变化的响应
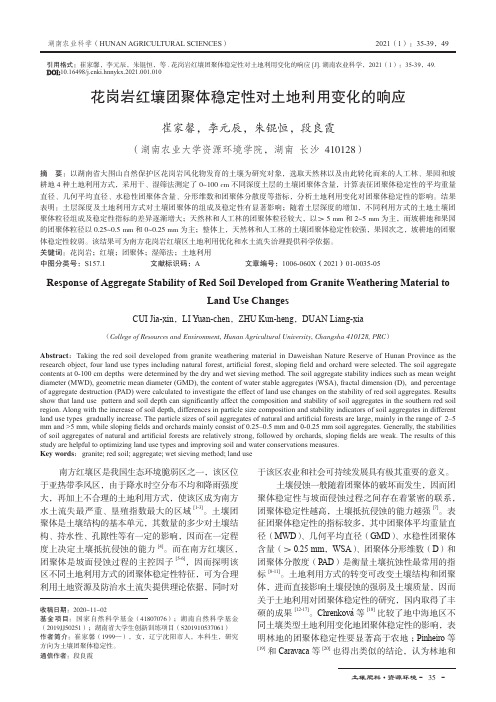
引用格式:崔家馨,李元辰,朱锟恒,等. 花岗岩红壤团聚体稳定性对土地利用变化的响应[J]. 湖南农业科学,2021(1):35-39,49. DOI:10.16498/ki.hnnykx.2021.001.010南方红壤区是我国生态环境脆弱区之一,该区位于亚热带季风区,由于降水时空分布不均和降雨强度大,再加上不合理的土地利用方式,使该区成为南方水土流失最严重、垦殖指数最大的区域[1-3]。
土壤团聚体是土壤结构的基本单元,其数量的多少对土壤结构、持水性、孔隙性等有一定的影响,因而在一定程度上决定土壤抵抗侵蚀的能力[4]。
而在南方红壤区,团聚体是坡面侵蚀过程的主控因子[5-6],因而探明该区不同土地利用方式的团聚体稳定性特征,可为合理利用土地资源及防治水土流失提供理论依据,同时对于该区农业和社会可持续发展具有极其重要的意义。
土壤侵蚀一般随着团聚体的破坏而发生,因而团聚体稳定性与坡面侵蚀过程之间存在着紧密的联系,团聚体稳定性越高,土壤抵抗侵蚀的能力越强[7]。
表征团聚体稳定性的指标较多,其中团聚体平均重量直径(MWD)、几何平均直径(GMD)、水稳性团聚体含量(>0.25 mm,WSA)、团聚体分形维数(D)和团聚体分散度(PAD)是衡量土壤抗蚀性最常用的指标[8-11]。
土地利用方式的转变可改变土壤结构和团聚体,进而直接影响土壤侵蚀的强弱及土壤质量,因而关于土地利用对团聚体稳定性的研究,国内取得了丰硕的成果[12-17]。
Chrenková等[18]比较了地中海地区不同土壤类型土地利用变化地团聚体稳定性的影响,表明林地的团聚体稳定性要显著高于农地;Pinheiro等[19]和Caravaca等[20]也得出类似的结论,认为林地和 花岗岩红壤团聚体稳定性对土地利用变化的响应 崔家馨,李元辰,朱锟恒,段良霞 (湖南农业大学资源环境学院,湖南长沙 410128)摘 要:以湖南省大围山自然保护区花岗岩风化物发育的土壤为研究对象,选取天然林以及由此转化而来的人工林、果园和坡耕地4种土地利用方式,采用干、湿筛法测定了0~100 cm不同深度土层的土壤团聚体含量,计算表征团聚体稳定性的平均重量直径、几何平均直径、水稳性团聚体含量、分形维数和团聚体分散度等指标,分析土地利用变化对团聚体稳定性的影响。
种衣营养剂与土壤改良剂处理瓜类种子对农药减量的影响
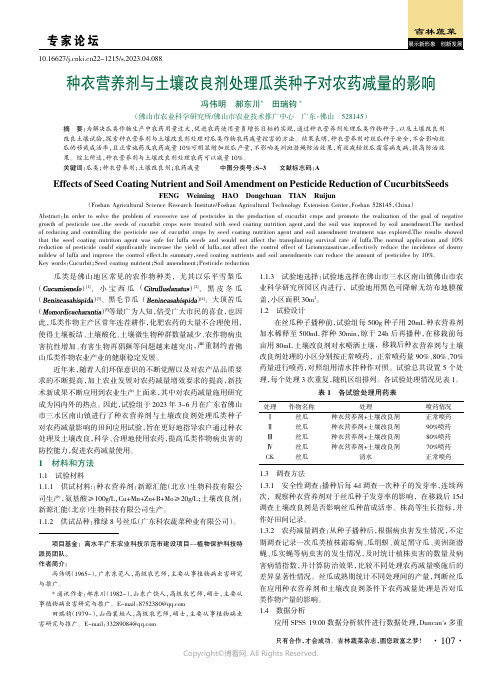
种衣营养剂与土壤改良剂处理瓜类种子对农药减量的影响冯伟明郝东川*田瑞钧*(佛山市农业科学研究所/佛山市农业技术推广中心广东窑佛山528145)摘要:为解决瓜类作物生产中农药用量过大,促进农药使用量负增长目标的实现,通过种衣营养剂处理瓜类作物种子,以及土壤改良剂改良土壤试验,探索种衣营养剂与土壤改良剂处理对瓜类作物农药减量控害的方法。
结果表明,种衣营养剂对丝瓜种子安全,不会影响丝瓜的移栽成活率,且正常施药及农药减量10%可明显增加丝瓜产量,不影响美洲斑潜蝇防治效果,有效减轻丝瓜霜霉病发病,提高防治效果。
综上所述,种衣营养剂与土壤改良剂处理农药可以减量10%。
关键词:瓜类;种衣营养剂;土壤改良剂;农药减量中图分类号:S-3文献标志码:AEffects of Seed Coating Nutrient and Soil Amendment on Pesticide Reduction of CucurbitsSeedsFENG Weiming HAO Dongchuan TIAN Ruijun(Foshan Agricultural Science Research Institute/Foshan Agricultural Technology Extension Center ,Foshan 528145,China )Abstract :In order to solve the problem of excessive use of pesticides in the production of cucurbit crops and promote the realization of the goal of negativegrowth of pesticide use ,the seeds of cucurbit crops were treated with seed coating nutrition agent ,and the soil was improved by soil amendment.The method of reducing and controlling the pesticide use of cucurbit crops by seed coating nutrition agent and soil amendment treatment was explored.The results showed that the seed coating nutrition agent was safe for luffa seeds and would not affect the transplanting survival rate of luffa.The normal application and 10%reduction of pesticide could significantly increase the yield of luffa ,not affect the control effect of Liriomyzasativae ,effectively reduce the incidence of downy mildew of luffa and improve the control effect.In summary ,seed coating nutrients and soil amendments can reduce the amount of pesticides by 10%.Key words :Cucurbit ;Seed coating nutrient ;Soil amendment ;Pesticide reduction项目基金:高水平广东农业科技示范市建设项目--植物保护科技特派员团队。
黄河三角洲土壤古菌群落结构对盐生植被演替的响应

黄河三角洲土壤古菌群落结构对盐生植被演替的响应张玥;孔强;郭笃发;金建玲;姜爱霞;郭健【摘要】This study attempted to investigate the soil archaeal community structure of bare board and other four kinds of halophyte vegetation (Saline Seepweed, Angiospermae, Imperata and A. venetum) in the Yellow River Delta by using high-throughput sequencing technology, and revealed its response to halophytic vegetation succession. The vegetation coverage would help to reduce the extent of soil salinization and increase the content of soil nutrients. The soil available phosphorus had a rising trend with the salt vegetation forward succession in bare board- heavy salt soil(Saline Seepweed, Angiospermae)- light salt soil(Imperata, A. venetum). The soil archaeal community structure of the 5soil differed significantly, bare board with no vegetation coverage had the highest soil archaeal community diversity and richness, and Euryarchaeota was the advantage microflora. Soil archaeal community structure wasn’t consistent strictly with halophytic vegetation succession. The community structure of soil archaea didn’t have obvious similarity when the halophytic vegetation succession at the same stage. Most community structure of soil archaea had differences at different successional stages, while the differences were not all greater when at the different stage. With the salt vegetation forward succession, the soil temperature, electrical conductivity (salinity) and total nitrogen played catalytic roles to soil archaeal community structure diversity, while the soil available nitrogen and organic matter hadinhibitory effects.%采用高通量测序技术,探究黄河三角洲光板地和4种典型盐生植被类型(翅碱蓬、獐茅、白茅和罗布麻)下土壤古菌群落组成和数量分布特征,揭示其对盐生植被演替的响应.结果表明,植被覆盖有利于降低土壤盐化程度、增加土壤营养物质含量,土壤速效磷随盐生植被在光板地-重盐土壤(翅碱蓬、獐茅)-轻盐土壤(白茅、罗布麻)中的正向演替有不断升高的趋势.5种覆被类型下土壤古菌在种群组成上差异明显,无植被覆盖的光板地具有最高的古菌群落多样性和丰富度,广古菌门为优势菌群.土壤古菌群落结构与盐生植被演替顺序不具有严格的一致性:当植被处于相同演替阶段时,土壤古菌群落结构不具明显的相似性;在不同演替阶段时,土壤古菌群落结构也未均表现出更大的差异性.随着盐生植被正向演替,土壤温度、电导率(含盐量)对土壤古菌群落结构多样性起促进作用,土壤全氮、碱解氮、有机质起抑制作用.【期刊名称】《中国环境科学》【年(卷),期】2016(036)007【总页数】7页(P2162-2168)【关键词】黄河三角洲;古菌群落结构;高通量测序;盐生植被【作者】张玥;孔强;郭笃发;金建玲;姜爱霞;郭健【作者单位】山东师范大学地理与环境学院,山东济南250014;山东师范大学地理与环境学院,山东济南250014;山东师范大学地理与环境学院,山东济南250014;山东大学生命科学学院,山东济南 250100;山东师范大学地理与环境学院,山东济南250014;山东农业工程学院食品科学与工程系,山东济南 250100【正文语种】中文【中图分类】X53古菌曾被认为是细菌的一个分支,直到Woese[1]于1990年首次将自然界微生物分为3大领域:真核生物、细菌和古菌,使古菌明显区别于细菌.在微生物非培养技术应用之前,古菌被认为只存在于热泉、盐湖和深海热液等极端环境中[2].近年来的研究表明,古菌广泛分布于土壤、湖泊、河流、海洋以及沼泽等环境中[3-6].由于极端的生存环境以及未知的生理过程和功能,古菌被现代微生物学、环境学等学科视为重要的研究对象之一[7],其在植物—土壤—古菌生态系统中的作用日渐凸显.研究古菌群落结构多样性有助于较早反映土壤质量变化,成为评价自然或人为干扰引起的土壤质量变化的重要指示因子[8].黄河三角洲为陆进海退之地,由于其离海距离、成陆时间及土壤盐碱程度不同,以裸地为起点,形成了强度、中度、轻度不同耐盐程度的植被演替系列[9],是研究盐碱土壤微生物群落及其与盐生植被演替关系的天然实验室.近年来对黄河三角洲微生物的研究主要集中于盐渍土壤或石油污染土壤中细菌群落结构方面[10-11],国内外对于古菌群落结构多样性的研究仍很薄弱,尤其对盐生植被演替过程中土壤古菌响应的研究还鲜见报道.与国内普遍使用的克隆测序技术相比,高通量测序技术拥有数据产出通量高、获得信息丰富等优点[12].本研究利用高通量测序技术研究黄河三角洲土壤古菌群落结构和数量分布特征,揭示其与植被演替的关系,明确暖温带滨海盐渍土区古菌微生物群落结构和数量分布特征,旨在为进一步研究滨海盐渍土区生物组成提供科学依据. 土壤样品于2012年6月采自黄河三角洲(现代三角洲),在研究区内沿东西向路线,依照盐生植被演替顺序,选择光板地、2种强耐盐植物群落(翅碱蓬群落、獐茅群落)、2种轻耐盐植物群落(白茅群落、罗布麻群落),采用对角线五点采样法,每个植物群落取3个样地,采集土壤样品.采样时,采集深度为0~20cm,并混合均匀,除去较大的根系等杂物,分为2份,每份约200g.一份土样封于灭菌袋中,于4℃条件下保存,用于土壤理化性质分析;另一份土样放入液氮罐带回实验室,于-80℃条件下保存,用于分子生物学研究[13].根据美国土壤质地分类制分析土壤质地,使用电导率仪(DDS-307,雷磁)测定土壤电导率,使用元素分析仪(vario Macro cube,德国Elementar)测定土壤全氮,采用碱解扩散法测定土壤碱解氮,土壤速效磷测定采用Olsen法,土壤有机质测定采用低温外加热重铬酸钾氧化-比色法[14].土壤样品DNA的提取采用OmegaDNA提取试剂盒(#D5625-01),依据试剂盒操作说明书进行操作.PCR扩增所用引物:正向F引物(CGTATCG- CCTCCCTCGCGCCATCAG+BACODE+PRIM-EF)、反向R引物(CTATGCGCCTTGCCAGCCC- GCTCAG+PRIMER).PCR反应体系的各组分组成:模板DNA 10.0ng,引物(50μmol/L)各1.0μL,10×PCR buffer 5.0μL,dNTP(10mmol/L)0.5μL, Plantium TaqÒDNA聚合酶0.5μL,ddH2O补至50.0μL.PCR热循环参数:94℃,预变性30s;94℃,变性20s;45℃,复性20s;65℃,延伸1min,扩增5个循环;94℃,变性20s;60℃,复性20s;72℃,延伸20s,扩增20个循环;72℃,延伸5min;10℃保存.对PCR产物进行琼脂糖电泳测定,采用上海生工琼脂糖回收试剂盒(cat:SK8131)对DNA进行回收.回收产物使用QubitÒ2.0荧光剂进行定量处理,将所有样品根据测得的DNA浓度按1:1混合,并充分震荡均匀.混合产物使用罗氏公司454测序仪(Roch GS FLX sequencer)进行测序.将测序所得序列与RDP数据库比对进行物种分类,通过Mothur软件分析,将相似性大于97%的序列归为同一种可操作分类单元(OTU),并进行α多样性分析,计算α多样性指数:丰富度指数(Richness)、香农指数(Shannon)、ACE指数、覆盖率(Coverage).土壤理化性质见表1.在5种覆被类型中,光板地、翅碱蓬地、白茅地属于粉砂壤土,獐茅地和罗布麻地均属于粉砂土.由于黄河三角洲土壤可溶性盐分主要为NaCl,土壤电导率与盐分含量可用线性方程模拟[15],采用土壤电导率表征土壤含盐量.5种土壤中光板地、獐茅地、翅碱蓬地为海积潮湿正常盐成土,白茅地和罗布麻地为弱盐淡色潮湿雏形土,有植被覆盖土壤盐化程度较小,无植被覆盖的光板地盐化程度最高,其电导率达1.95dS/m.有机质、全氮、碱解氮在有植被覆盖土壤中的含量均高于光板地.速效磷含量白茅和罗布麻地高于翅碱蓬和獐茅地,光板地含量最低.可以看出,植被覆盖有利于降低土壤盐化程度、增加土壤营养物质含量,土壤速效磷随盐生植被在光板地-重盐土壤(翅碱蓬、獐茅)-轻盐土壤(白茅、罗布麻)中的正向演替有不断升高的趋势.高通量测序结果经过低质量序列过滤后,得到的序列长度大部分分布于400~600bp之间,平均长度在440bp以上,满足了进一步分析的质量要求.5个土壤样本共获得80874条有效序列,分别为:光板地9586条,翅碱蓬11030条,獐茅23263条,白茅22794条,罗布麻14201条.OTU统计结果如表2所示.5种覆被类型下的土壤古菌序列主要归属于两个门类:泉古菌门(Crenarchaeota)和广古菌门(Euryarchaeota).广古菌门在五种土壤中的相对丰度为97.15%(光板地)~4.63%(白茅土壤),为优势菌群;泉古菌门在5种土壤中的相对丰度比广古菌门小得多,仅为1.61%(白茅土壤)~ 0.05%(翅碱蓬土壤);未分类门在五种土壤中的相对丰度为93.75%(白茅土壤)~2.77%(光板地),差异较大.检测到4个纲分类单元:广古菌门下的盐杆菌纲(Halobacteria)、热原体纲(Thermoplasmata)、甲烷微菌纲(Methanomicrobia),泉古菌门下的热变形菌纲(Thermoprotei),其中盐杆菌纲为优势菌群;未分类纲在五种土壤中的相对丰度为98.04%(白茅土壤)~9.55%(光板地),差异较大.五种覆被类型下共检测到26个属分类单元,除热裸单胞菌属(Thermogymnomonas)属于热原体纲下的热原体目(Thermoplasmatales)–Thermoplasmatales_incertae_sedis外,其余古菌属均属于盐杆菌纲下的盐杆菌目(Halobacteriales)–盐杆菌科(Halobacteriaceae),各古菌属相对丰度具体情况如图1所示,其中Halolamina、盐红菌属(Halorubrum)、盐盒菌属(Haloarcula)为优势菌群,光板地中古菌属种类最多,罗布麻土壤次之,白茅土壤最少;未分类属在5种土壤中的相对丰度为99.89%(白茅土壤)~79.43%(光板地).经相关性分析发现,广古菌门整体与土壤温度、含水率和电导率呈正相关关系,与土壤全氮、碱解氮、速效磷和有机质呈负相关关关系,其中甲烷微菌目(Methanomicrobiales)与土壤温度的相关系数为0.959(P<0.01),呈极显正著相关,说明该菌目对土壤温度敏感,与土壤碱解氮的相关系数为-0.926(P<0.05),呈显著负相关; 各古菌属与土壤理化性质的正负相关关系不完全相同,其中盐碱团球菌属(Halalkalicoccus)、盐长命菌属(Halovivax)、盐红菌属、盐棍菌属(Halorhabdus)、富盐菌属(Haloferax)、盐几何菌属(Halogeometricum)、盐陆生菌属(Haloterrigena)、盐盒菌属、盐简菌属(Halosimplex)与土壤电导率的相关系数分别为0.986、0.986、0.978、0.986、0.963、0.986、0.962、0.980、0.979(P<0.01),均呈极显著正相关,说明这些稀有菌属均为嗜盐菌属或耐盐菌属.泉古菌门整体与土壤温度、电导率呈负相关关系,与其余土壤理化性质呈正相关关系,其中与土壤全氮的相关系数为0.900(P<0.05),为显著正相关.RDA排序结果如图2所示.第1轴序(Axis1)与第2轴序(Axis2)共可解释86.64%的变量.土壤温度、含水量、土壤电导率(含盐量)与大部分古菌属呈正相关关系;土壤全氮、碱解氮、速效磷、有机质与大部分古菌属呈负相关关系.土壤温度、电导率(含盐量)、全氮、碱解氮、有机质箭头相对较长,可得这5种土壤理化性质对土壤古菌属群落结构多样性影响较大,且土壤温度、电导率(含盐量)对大部分古菌生长起促进作用,土壤中的营养物质反而会抑制大部分古菌的生长.卡方数值越大,植被间古菌群落结构多样性差异越大.从表3的卡方检验结果可知,土壤古菌群落结构对盐生植被演替表现出一定程度的响应,但各古菌属未呈现统一的随盐生植被演替增加或减少的规律:光板地下的古菌群落结构与有植被覆盖的翅碱蓬、獐茅、白茅、罗布麻土壤下的均呈现不同程度的差异性,与属轻盐土壤的白茅土壤显著性差异最大,与重盐土壤(獐茅、白茅)次之,与属轻盐土壤的罗布麻土壤显著性差异最小,且罗布麻土壤中除盐碱团球菌属外其他古菌属的相对丰度皆比光板地中的极显著增加;重盐土壤(獐茅、白茅)下的古菌群落结构整体与轻盐土壤(白茅、罗布麻)下的呈现差异性,重盐土壤(獐茅、白茅)整体土壤古菌属与罗布麻土壤显著性差异最大,且相对丰度均比罗布麻土壤中的极显著减少;重盐土壤(獐茅、白茅)之间,古菌群落结构多样性差异较小;轻盐土壤(白茅、罗布麻)之间,古菌群落结构多样性呈现差异性,且白茅土壤中的大部分古菌相对丰度比罗布麻土壤中的极显著减少. 注: ** 为P < 0.01极显著差异,*为0.01 £P < 0.05显著差异;—为不符合卡方检验要求.本研究中,广古菌门在5种所研究的覆被下为优势菌群,泉古菌门相对丰度要小得多,这与以往利用分子生物学方法对盐渍环境古菌样品进行分析研究所获得的结果相似.比如,Youssef等[16]采用16S rRNA方法对盐渍沉积物进行研究,发现超过60%的古菌序列归属于广古菌门的盐杆菌纲.广古菌门中,除极少量甲烷微菌纲和热源体纲,5种土壤中检测到的26个古菌属中有25个(96.15%)属于盐杆菌纲中的盐杆菌目、盐杆菌科,为嗜盐古菌.嗜盐古菌最显著的特征是其需要独特的生长环境:对高浓度NaCl依赖性强,至少需要1.5mol/L的NaCl才能生长,最适NaCl生长浓度为3.5~4.5mol/L[17].目前通过分离得到的嗜盐古菌大多来自高盐环境,如海洋、盐(碱)湖、晒盐场、高盐浓度土壤、人工盐制品等.Walsh等[18]采用16S rRNA方法对盐泉岛古菌多样性进行调查,发现嗜盐古菌占70%以上,并随着盐浓度的升高,多样性增加.与Walsh研究结论相似,本文在土壤盐分含量最高的光板地中,嗜盐古菌多样性和相对丰度均最高,其中21个属(占光板地所含古菌属总量的84%)属于嗜盐古菌;属于轻盐土壤的白茅土壤与其差异明显,只有5个属(占白茅土壤所含古菌属总量的20%)属于嗜盐古菌;但同属于轻盐土壤的罗布麻土壤中含有20个属(占罗布麻土壤所含古菌属总量的80%)属于嗜盐古菌,仅次于光板地中的含量.轻盐土壤中嗜盐古菌含量较高的原因有待进一步研究,也许与其好氧(或兼性厌氧)及生长所需的复杂营养物质有关.由图1可得,Halolamina在5种土壤中相对丰度最高,盐红菌属、盐盒菌属、盐粒菌属(Halogranum)、盐微菌属(Halomicrobium)、盐盘菌属(Haloplanus)、盐杆菌属(Halobacterium)、盐棒菌属(Halorhabdus)、Salarchaeum、盐碱单胞菌属(Natronomonas)相对丰度依次递减,其余嗜盐古菌相对丰度均不足1%.盐红菌属为嗜盐好氧古菌,栖息于盐渍或超盐渍土壤中[19];盐碱单孢菌属在盐碱环境中广泛存在[20],且生长需要异常高温[21],表明该古菌生理学特征较为独特.由表3可知,以上2个菌属在4种土壤中均比光板地中极显著减少,与其嗜盐特性对应.盐球菌属(Halococcus)发现于阿拉伯湾盐生植物盐节木的根际中,该古菌有降解石油的功能[22],本实验中只在罗布麻土壤中发现,日后可深入研究并加以利用.根据嗜盐古菌能否生长的pH值条件,可将其分为中性嗜盐古菌和嗜盐嗜碱古菌2类,但目前对嗜盐嗜碱古菌的研究十分有限.截止到目前发现的32个属于嗜盐古菌的属中,有7个属于嗜盐嗜碱菌属(盐碱杆菌属(NatronobacteriumTinball)、盐碱球菌属(Natronococcus Tinball)、盐碱单胞菌属、盐碱红菌属(Natronorubrum) 、盐碱团球菌属、盐碱湖菌属、盐碱古菌属(Natronoarchaeum)),在3个中性嗜盐菌(盐双形菌属(Halobiforma)、盐红菌属、盐无色菌属(Natrialba)中也有嗜盐嗜碱菌的存在.本文中检测到的嗜盐嗜碱菌包括盐碱团球菌属、盐碱古菌属、盐碱单胞菌属和盐盘菌属,除盐碱古菌属在罗布麻土壤中含量最高,其余三者均在光板地中含量最高.目前嗜盐嗜碱菌的分类主要依据16S rRNA序列的分析,随着研究范围的扩大、研究方法的改进,更多的嗜盐嗜碱菌属及其生理特性将得以发现和确认.本文中5种覆被类型下泉古菌门比广古菌门相对丰度小得多,且在泉古菌门中只检测到热变形菌纲的存在,与以往土壤古菌研究结果不同,这可能与黄河三角洲盐碱土壤本身性质及其中包含的其他微生物不适宜泉古菌门生长有关.比如,袁超磊等[23]利用454高通量测序技术对湖南湘阴县一典型红壤剖面进行研究,发现古菌以泉古菌门中的热变形菌纲(89%)为主,且其分布与土壤黏粒含量相关.Nicol等[24]利用16S rRNA方法对亚马逊4个不同地点的草地生态系统进行研究,发现检测到的泉古菌数量远远超过广古菌;Jackson等[25]通过采用16S rRNA方法对森林泥炭土的剖面进行分析,发现表层土壤中(0~10cm)未检测到古菌菌群,但深层土壤中(20~50cm)获得了泉古菌序列,未检测到广古菌序列;Chen等[26]利用分子生物学方法在水稻土中发现根际的氨氧化古菌(属于泉古菌门)数量高于非根际和未种植的水稻田,表明水稻根系一定程度上改变了泉古菌的数量且对其群落结构产生影响.本文中泉古菌门与土壤全氮呈显著正相关,可能与其在土壤中的氨氧化作用有关[27].4.1 植被覆盖有利于降低土壤盐化程度、增加土壤营养物质含量,土壤速效磷随盐生植被正向演替有不断升高的趋势.4.2 无植被覆盖的光板地具有最高的古菌群落多样性和丰富度,土壤古菌群落结构多样性与盐生植被演替顺序的响应不具有严格的一致性:当植被处于相同演替阶段时,土壤古菌群落结构不具明显的相似性;在不同演替阶段时,土壤古菌群落结构也未均表现出更大的差异性.4.3 土壤理化性质中的温度、电导率(含盐量)、全氮、碱解氮、有机质对土壤古菌属群落结构多样性影响较大.随着盐生植被正向演替,土壤温度、电导率(含盐量)对土壤古菌群落结构多样性起促进作用,土壤全氮、碱解氮、有机质起抑制作用. Woese C R, Kandler O, Wheelis M L. Toward anaturalsystem of organisms: Proposal for the domains archaea, bacteria and eucarya [J].Proc Natl Acad Sci USA, 1990,87:4576-4579.李曙光,皮昀丹.古菌研究及其展望 [J]. 中国科学技术大学学报, 2007,37(8):830-838.Damon C, Lehembre F, Oger-Desfeux C, et al. Metatranscriptomics reveals the diversity of genes expressed by eukaryotes in forest soils [J]. PloSone, 2012,7(1):e28967.Moran M A. Metatranscriptomics: eavesdropping oncomplex microbial communities [J]. Microbe, 2009,4(7):329-335.武传东,闫倩,辛亮,等.长期施用氮肥和磷肥对渭北旱塬土壤中氨氧化古菌多样性的影响 [J]. 农业环境科学学报, 2012,4:743-749.周磊榴,祝贵兵,王衫允,等.洞庭湖岸边带沉积物氨氧化古菌的丰度、多样性及对氨氧化的贡献 [J]. 环境科学学报, 2013,6: 1741-1747.曹鹏,沈菊培,贺纪正.古菌细胞膜脂在古菌群落组成及其对环境响应研究中的应用[J]. 应用生态学报, 2012,9:2609-2616.林先贵,胡君利.土壤微生物多样性的科学内涵及其生态服务功能 [J]. 土壤学报, 2008,45(5):892-900.郭笃发.黄河三角洲土地利用/土地覆被时空变化研究 [D]. 沈阳:沈阳农业大学, 2004.Wang Z Y, Xin D M, Gao F M, et al. Microbialcommunity characteristics in a degraded wetland of the Yellow River Delta [J].Pedosphere, 2010,20(4):466-478.Yu S L, Li Y Q, TangX L, et al. Succession of bacterial community along with the removal of heavycrude oil pollutants by multiple biostimulation treatments in the Yellow RiverDelta, China [J]. Journal of Environmental Sciences, 2011,23(9):1533-1543. 何芝,赵天涛,邢志林,等.典型生活垃圾填埋场覆盖土微生物群落分析 [J]. 中国环境科学, 2015,35(12):3744-3753.何苑皞,周国英,王圣洁,等.杉木人工林土壤真菌遗传多样性 [J]. 生态学报, 2014,10(10):2725-2736.鲍士旦.土壤农化分析 [M]. 北京:中国农业出版社, 2000.翁永玲,官鹏.黄河三角洲盐渍土盐分特征研究 [J]. 南京大学学报(自然科学), 2006,42(6):602-610.吴尊凤,史应武,娄恺,等.天山北坡垂直自然带土壤古菌多样性分析[J]. 新疆农业科学, 2012,3:488-495.刘冰冰,唐蜀昆,明红,等.新疆罗布泊地区可培养嗜盐古菌多样性及其功能酶筛选[J]. 微生物学报, 2011,9:1222-1231.Walsh D A, Papke R T, Doolittle W F. Archaealdiversity along a soil salinity gradient prone to disturbance [J]. Environmental Microbiolofy, 2005,7:1655-1666.Papke R T, Koenig J E, Rodriguez-Valera F, et al. Frequent recombination ina salternpopulation of Halorubrum [J]. Science, 2004,306:1928–1929.Valenzuela-Encinas C, Neria-Gonzalez I,Alcantara-Hernandez R J, et al.Phylogenetic analysis of the archaeal community in an alkaline-saline soil of theformer lake Texcoco (Mexico) [J]. Extremophiles, 2008,12:247–254.Inoue K, Itoh T, Ohkuma M, et al. Halomarina oriensisgen. nov., sp. nov., a halophilic archaeon isolated from aseawater aquarium [J]. Int. J. Syst. Evol. Microbiol., 2011,61:942–946.Al-Mailem D M, Sorkhoh N A, Marafie M, et al. Oil phytoremediation potential of hypersaline coasts of theArabian Gulf using rhizosphere technology [J]. Bioresour. Technol., 2010,101:5786–5792.袁超磊,贺纪正,沈菊培,等.一个红壤剖面微生物群落的焦磷酸测序法研究 [J]. 土壤学报, 2013,1:138-149.Nicol G W, Leininger S, Schleper C, et al. Theinfluence of soilpH on the diversity, abundance and transcriptional activity ofammonia oxidizing archaea and bacteria [J]. Environ. Microbiol.,2008,10:2966-2978.Jackson C R, Liew K C, Yule C M. Structural andfunctional changes with depth in microbial communities in a tropical peat swampforest [J]. Microbiol. Ecol., 2008, online.Chen X P, Zhu Y G, Xia Y, et al. Ammonia-oxidizing archaea:important players in paddy rhizosphere soil [J]. Environ. Microbiol., 2008,10:1978-1987.鲍林林,陈永娟,王晓燕.北运河沉积物中氨氧化微生物的群落特征 [J]. 中国环境科学, 2015,35(1):179-189.* 责任作者, 教授,***************。
土壤质地对小麦SPS、SS活性及其与淀粉合成关系的影响
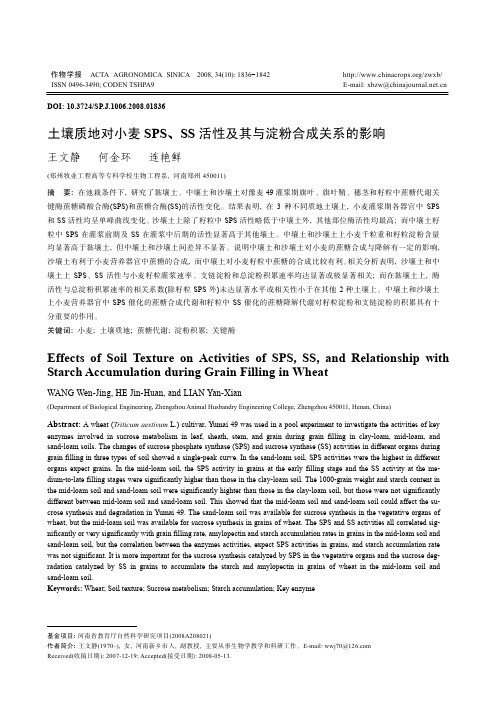
作物学报ACTA AGRONOMICA SINICA 2008, 34(10): 1836-1842http:///zwxb/ ISSN 0496-3490; CODEN TSHPA9E-mail: xbzw@DOI: 10.3724/SP.J.1006.2008.01836土壤质地对小麦SPS、SS活性及其与淀粉合成关系的影响王文静何金环连艳鲜(郑州牧业工程高等专科学校生物工程系, 河南郑州450011)摘要: 在池栽条件下, 研究了黏壤土、中壤土和沙壤土对豫麦49灌浆期旗叶、旗叶鞘、穗茎和籽粒中蔗糖代谢关键酶蔗糖磷酸合酶(SPS)和蔗糖合酶(SS)的活性变化。
结果表明, 在3种不同质地土壤上, 小麦灌浆期各器官中SPS和SS活性均呈单峰曲线变化。
沙壤土上除了籽粒中SPS活性略低于中壤土外, 其他部位酶活性均最高; 而中壤土籽粒中SPS在灌浆前期及SS在灌浆中后期的活性显著高于其他壤土。
中壤土和沙壤土上小麦千粒重和籽粒淀粉含量均显著高于黏壤土, 但中壤土和沙壤土间差异不显著。
说明中壤土和沙壤土对小麦的蔗糖合成与降解有一定的影响,沙壤土有利于小麦营养器官中蔗糖的合成, 而中壤土对小麦籽粒中蔗糖的合成比较有利。
相关分析表明, 沙壤土和中壤土上SPS、SS活性与小麦籽粒灌浆速率、支链淀粉和总淀粉积累速率均达显著或极显著相关; 而在黏壤土上, 酶活性与总淀粉积累速率的相关系数(除籽粒SPS外)未达显著水平或相关性小于在其他2种土壤上。
中壤土和沙壤土上小麦营养器官中SPS催化的蔗糖合成代谢和籽粒中SS催化的蔗糖降解代谢对籽粒淀粉和支链淀粉的积累具有十分重要的作用。
关键词:小麦; 土壤质地; 蔗糖代谢; 淀粉积累; 关键酶Effects of Soil Texture on Activities of SPS, SS, and Relationship with Starch Accumulation during Grain Filling in WheatWANG Wen-Jing, HE Jin-Huan, and LIAN Yan-Xian(Department of Biological Engineering, Zhengzhou Animal Husbandry Engineering College, Zhengzhou 450011, Henan, China)Abstract: A wheat (Triticum aestivum L.) cultivar, Yumai 49 was used in a pool experiment to investigate the activities of key enzymes involved in sucrose metabolism in leaf, sheath, stem, and grain during grain filling in clay-loam, mid-loam, and sand-loam soils. The changes of sucrose phosphate synthase (SPS) and sucrose synthase (SS) activities in different organs during grain filling in three types of soil showed a single-peak curve. In the sand-loam soil, SPS activities were the highest in different organs expect grains. In the mid-loam soil, the SPS activity in grains at the early filling stage and the SS activity at the me-dium-to-late filling stages were significantly higher than those in the clay-loam soil. The 1000-grain weight and starch content in the mid-loam soil and sand-loam soil were significantly highter than those in the clay-loam soil, but those were not significantly different between mid-loam soil and sand-loam soil. This showed that the mid-loam soil and sand-loam soil could affect the su-crose synthesis and degradation in Yumai 49. The sand-loam soil was available for sucrose synthesis in the vegetative organs of wheat, but the mid-loam soil was available for sucrose synthesis in grains of wheat. The SPS and SS activities all correlated sig-nificantly or very significantly with grain filling rate, amylopectin and starch accumulation rates in grains in the mid-loam soil and sand-loam soil, but the correlation between the enzymes activities, expect SPS activities in grains, and starch accumulation rate was not significant. It is more important for the sucrose synthesis catalyzed by SPS in the vegetative organs and the sucrose deg-radation catalyzed by SS in grains to accumulate the starch and amylopectin in grains of wheat in the mid-loam soil and sand-loam soil.Keywords: Wheat; Soil texture; Sucrose metabolism; Starch accumulation; Key enzyme基金项目:河南省教育厅自然科学研究项目(2008A208021)作者简介:王文静(1970-), 女, 河南新乡市人, 副教授, 主要从事生物学教学和科研工作。
- 1、下载文档前请自行甄别文档内容的完整性,平台不提供额外的编辑、内容补充、找答案等附加服务。
- 2、"仅部分预览"的文档,不可在线预览部分如存在完整性等问题,可反馈申请退款(可完整预览的文档不适用该条件!)。
- 3、如文档侵犯您的权益,请联系客服反馈,我们会尽快为您处理(人工客服工作时间:9:00-18:30)。
Soil Contributions to Blasting Effects from Case Histories and Vibration Limits Mark R. Svinkin, Member, ASCE 1 1VIBRACONSULT, 13821 Cedar Road, #205, Cleveland, OH 44118-2376; PH (216) 397-9625; FAX (216) 397-9625; msvinkin@ Abstract The existing vibration limits were developed for 1-2 story houses surrounding coal surface mines, and these limits are applied for ground vibrations as the criteria for the possible crack formation in structures. However, other blast design, soil conditions and structures require different vibration limits. There are no regulations for multistory building vibrations from blasting and there are no limits to assess possible dynamic settlements of soil and structures triggered by ground vibrations from blasting. Nevertheless, numerous case histories are available in technical papers which describe tolerable and unacceptable soil and structural vibrations measured for different soil conditions and structures. These case histories are valuable sources of information to evaluate vibration effects on structures, analyze vibration levels that do not produce damage to structures, determine the areas of application of the existing regulations and assess tolerable vibration limits for various structures during blasting operations. Introduction Blasting operations conducted in urban areas are usually connected with potential problems of manifestation of vibration effects on adjacent and remote houses, buildings and other super and underground structures. Dynamic consequences of structural vibrations range from benign to harmful. It is common that two significant questions come up at neighborhood areas around sites with blasting operations: what is the cause of damage to structures and what vibration limits have to be used at specified areas. It is necessary to underline that similar damage to structures can be produced by different group of static and dynamic loads such as environmental forces (temperature, humidity, wind, rain, snow, etc.), geotechnical hazards (heave, settlement, stability, sliding and failure), and dynamic effects from blasting. Determination of actual causes of damage to structures withoutthe performance of a pre- and post-blast structure surveys is a complicated task, and 335D o w n l o a d e d f r o m a s c e l i b r a r y .o r g b y O c e a n U n i v e r s i t y o f C h i n a o n 11/19/13. C o p y r i g h t A S CE .F o r p e r s o n a l u s e o n l y ; a l l r i g h t s r e s e r v e d .missing surveys are the basis for contradictory judgments of blasters and house owners. Counteraction between both sides sometimes extends in large scales. On the one hand blasters stand up for their correct blasting operations without any damage to structures. On the other hand residents who believe that their houses were damaged by blasting joint efforts to push forward the legislature for diminishing the vibration limits, increase areas of pre-blasting survey and choose larger scaled-distances to decrease vibration effects on houses from blasting. Such restrictions can create substantial obstacles for production blasts without insurance of safe blasting. Obviously, a number of resident complaints are related to public perception of blasting and public relations. However, there are case histories with real damage to structures from blasting. Vibration limits correctly determined can help to properly assess vibration problems during blasting. Case histories are large and valuable sources of information which can be used to determine vibration limits. In this paper, a number of case histories are analyzed with an emphasis on soil contributions to blasting effects on wave propagation and damage to structures. The Existing Vibration Limits Apparently, it is impossible to have universal regulations of blasting vibrations applied for different kinds of blasting, variety of soil conditions and types of structures. The available optional regulations, NFPA 495 (2006), include the vibration cosmetic cracking thresholds for assessment of surface coal mine blasting effects on 1-2 story residential houses, Figure 1. These criteria developed by the U.S. Bureau of Mines (USBM) have the following displacement and velocity values for the four ranges of the dominant frequencies: 0.76 mm (0.03 in) for 1-4 Hz, 19 mm/s (0.75 in/s) for 4-15 Hz, 0.2 mm (0.008 in) for 15-40 Hz, and 50.8 mm/s (2.0 in/s) for 40-100 Hz. The limit of 19 mm/s (0.75 in/s) for 4-15 Hz is used for drywall while the limit of 13 mm/s (0.5 in/s) for 2.5-10 Hz is applied for plaster, Siskind et al. (1980). The derivative version of the USBM safe limits shown in Figure 1 was included as the Chart Option into the surface coal mine regulations by the Office of Surface Mining Reclamation and Enforcement, OSM (1983). The USBM vibration limits were built up on the basis of the two decades research studies of a correlation between ground vibrations and observations of blast damage to low-rise houses, and these limits are applied for ground vibrations as the criteria of possible crack formation in various structures. The USBM research study has been recognized as the great achievement which provides the safety of 1-2 story residential houses from vibrations generated by surface coal mining blasting. Obviously, these vibration limits can be successfully used for adequate blasting loads, similar structures and ground conditions they were developed for, but different limits should be used for other combination of dynamic loads, soil conditions and structures.FORENSIC ENGINEERING 2009336D o w n l o a d e d f r o m a s c e l i b r a r y .o r g b y O c e a n U n i v e r s i t y o f C h i n a o n 11/19/13. C o p y r i g h t A S C E . F o r p e r s o n a l u s e o n l y ; a l l r i g h t s r e s e r v e d .Figure 1. Safe level blasting criteria from USBM RI 8507 and the derivative version, the Chart Option from OSM surface coal mine regulations. Shaded area shows maximum velocities of resonant structural vibrations with amplification of 4.5. Data were modified from Siskind (2000). Plot was adapted from Svinkin (2008). Siskind (2000) presented the accumulated results of research studies accomplished by the USBM and others on vibrations from blasting and their effects on low-rise houses, Figure 2. Ground vibrations were measured near 1-2 story residential structures from 718 blasts and 233 documented observations were obtained. Non-damaging blasts are not shown although some of them produced relatively high level of ground vibrations even exceeding 51 mm/s (2 in/s). These data indicate different vibration effects on structures depending on the dominant frequency and the peak particle velocity (PPV) of ground vibrations. It is necessary to point out that the USBM criteria are conservative for assessment of direct blasting vibration effects on structures in the non-resonant frequency zones of structural vibrations when ground vibrations do not trigger plastic soil deformations under structures (Figure 2), but they cannot protect low-rise structures from appearance of cosmetic cracks by amplification of ground vibrations higher than 4.5x and beyond the 4-12 Hz frequency range, Svinkin (2003, 2004). Furthermore, the application of these limits to different super and underground structure is incorrect. AASHTO Designation: R 8-96 (2004) stated the application of the USBM limits to markedly different types of structures is common and inaccurate. Besides, the USBM limits do not actually take into account construction blasts with much higher frequency content. Dowding (1996) demonstrated examples where the dominant frequencies of ground vibrations from surface mining and construction blasting would lie between 12 and 18 Hz and 70 and 100 Hz, respectively. It means that the USBM limits cannot beused for construction blasts.FORENSIC ENGINEERING 2009337D o w n l o a d e d f r o m a s c e l i b r a r y .o r g b y O c e a n U n i v e r s i t y o f C h i n a o n 11/19/13. C o p y r i g h t A S CE .F o r p e r s o n a l u s e o n l y ; a l l r i g h t s r e s e r v e d .Some publications have stated that there are no problems with blasting vibration limits. For example, Aimone-Martin (2005) has asserted, that the safe blasting criteria defined by the U.S. Bureau of Mines in 1980 are widely used in the U.S. rock blasting industry. “This safe criteria is based on over 40 years of research and crack observations and has been scientifically supported since 1980 by experts in the field of structure response to rock blasting. There has been no scientific data to date that disputes this criteria.” On the one hand this statement is correct because the USBM criteria without doubt are good for the specific blast design, soil conditions and the types of structures they were developed for. On the other hand this statement is confusing because in a number of cases, these vibration limits are used for different blast, soil and structure conditions. Verification is necessary for the application of theUSBM criteria for other blasts, soil and 1-2 story houses. Figure 2. Ground vibrations from blasting and structural damage summary grouped in three zones. Dashed lines define USBM safe limits. Data were modified from Siskind (2000). Plot was adapted from Svinkin (2008). Siskind (1991) wrote, “Vibration criteria for nonresidential cases are needed: concrete, power poles, pipelines, bridges, etc. Without reliable criteria for such cases, regulation is often based on relatively strict levels required to prevent cosmetic damage to residences”. Also, Siskind (2000) stated that most regulatory bodies, including OSM, recognize “that a more rigorous treatment may be needed in special cases, such as that outlined in RI 8507”. Some states use single vibration limits between 6.4-51 mm/s (0.25-2.0 in/s) for PPV of ground vibrations that only aggravates problems with assessment of damage to houses because of lack of objectivity and ignorance of possible resonant structural vibrations. The British Standard BS 7385 (1993) and the USBM criteria are depicted in Figure 3. It can be seen that the BS 7385 considers two lines of the safe limits depending on a type of buildings. Line 1 with the frequency-independent criterion of 51 mm/s (2 in/s) isapplied to industrial and heavy commercial buildings. Line 2 with the frequency-based safe limits is similar to the USBM criteria and applied to residential or light commercial FORENSIC ENGINEERING 2009338D o w n l o a d e d f r o m a s c e l i b r a r y .o r g b y O c e a n U n i v e r s i t y o f C h i n a o n 11/19/13. C o p y r i g h t A S C E . F o r p e r s o n a l u s e o n l y ; a l l r i g h t s r e s e r v e d .type buildings. It seems the values presented in Figure 3 are small to represent structural vibrations. On the basis of similarity of two standards, it can be assumed that the BS 7385 requires measurement of ground vibrations as the criteria of possible structural damage. If so, the BS 7385 has similar restrictions of its application as the USBM criteria, Svinkin (2007).Figure 3. Vibration guidelines - USBM RI 8507 (solid line) compared to BS 7385 (dashed line). Line 1: reinforced or framed structures, industrial and heavy commercial buildings; line 2: unreinforced or light framed structures, residential or light commercial type buildings. From AASHTO Designation: R 8-96 (2004). The German Standard DIN 4150 (1986) and the USBM criteria are depicted in Figure 4. DIN 4150 suggests three lines of time-dependent vibration limits for different types of structures. Lines 1-3 represent different kinds of structures described in the figure caption of Figure 4. Lines 2 and 3 are located below the USBM vibration limits. Line 1 is close to the upper boundary of the USBM vibration limits in the 4-100 Hz frequency range. Comments are needed regarding structures with particular sensitivity to vibrations. Such terminology is appropriate for devices and technology sensitive to vibration, but structure sensitivity is different. Resonant vibrations are dangerous for any kind of structures: from buildings under the preservation orders to industrial buildings. Dynamic settlements can also be devastated for any kind of buildings. However, industrial buildings have much higher resistance to direct vibration effects than 1-2 story residential houses. The basis for the guideline level of DIN 4150 is unknown, AASHTO Designation: R 8-96 (2004). Obviously, these criteria intended to minimize human perceptions and complaints. This approach would be sensible if construction operationsFORENSIC ENGINEERING 2009339D o w n l o a d e d f r o m a s c e l i b r a r y .o r g b y O c e a n U n i v e r s i t y o f C h i n a o n 11/19/13. C o p y r i g h t A S CE .F o r p e r s o n a l u s e o n l y ; a l l r i g h t s r e s e r v e d .constantly affect houses and even at night. However, if blasts are performed 1-2 times per day, such an approach is unreasonable, Svinkin (2007).Figure 4. Vibration guidelines - USBM RI 8507 (solid line) compared to DIN 4150 (dashed line). Line 1: buildings used for commercial purposes, industrial buildings and buildings of similar design; line 2: dwellings and buildings of similar design and/or use; line 3: structures that because of their particular sensitivity to vibration, do not correspond to those listed in lines 1 and 2, and are of great intrinsic values (e.g. building that are under a preservation order). From AASHTO Designation: R 8-96 (2004). The Russian limits of 30-50 mm/s (1.18-1.97 in/s) for vibrations of sound structures were found by the Moscow Institute of Physics of the Earth to assess the safety of structures from explosive effects of various blasts in the air, on the ground and under the ground at the time of the Second World War, Sadovskii (1946). So, for multi-story residential, commercial and industrial buildings, the frequency-independent safe limit of 51 mm/s (2 in/s) can be chosen for the PPV of structural, not ground, vibrations. This criterion automatically takes into account soil-structure interaction for the whole building frequency range. In the support of the proposed criterion, it is necessary to underline that according to Siskind (2000), the PPV of 51 mm/s (2 in/s) is the highest vibration level generated inside homes by walking, jumping, slamming doors, etc. Besides, this vibration limit is compatible with the European Standards, and it does not exclude higher allowable vibration levels. It is easy to demonstrate compatibility of the new simplified safe criterion and the USBM vibration limits, Figure 1. To evaluate tolerable structural vibrations, the smallest vibration limits of 13 mm/s (0.5 in/s) and 19 mm/s (0.75 in/s) have to be multiplied by 4.5, and theirFORENSIC ENGINEERING 2009340D o w n l o a d e d f r o m a s c e l i b r a r y .o r g b y O c e a n U n i v e r s i t y o f C h i n a o n 11/19/13. C o p y r i g h t A S C E . F o r p e r s o n a l u s e o n l y ; a l l r i g h t s r e s e r v e d .products of 57 mm/s (2.25 in/s) and 85.5 mm/s (3.37 in/s) are higher than the simplified criterion of 51 mm/s (2 in/s), Svinkin (2007, 2008). Soil and Structure Responses to Blasting Blasting operations generate different body and surface waves which travel through the medium of soil deposits and rock. Elastic waves travel from dynamic sources and induce elastic soil deformations (ground vibrations) which levels depend on intensity of propagated waves. The structural responses to ground vibrations depend on soil-structure interaction. Ground vibrations can produce direct vibration effects on structures and trigger resonant structural vibrations of adjacent and remote structures. However, under certain circumstances such as a combination of non-cohesive soil deposits, water table levels and ground vibrations, elastic waves can be the cause of plastic soil deformations, e.g. liquefaction, densification and soil settlements triggered by small ground vibrations. Soil-structure interaction will be different for soil failure. The structural response to ground excitation depends on the soil response to waves propagated from the source and soil soil-structure interaction. Because elastic and plastic soil deformations cause dissimilar structural responses and damage, diverse thresholds have to be used for assessment of direct vibration effects, resonant structural vibrations, and plastic soil deformations (Svinkin, 2008). The existing regulations were developed for assessment of elastic soil deformations and structural responses to ground vibrations from blasting. These criteria have nothing to do with plastic soil deformations. It is necessary to underline that there are no federal, state and local regulations of the critical vibration levels of ground vibrations which may trigger dynamic soil failure. Therefore, an experience from cases histories of soil failure contribution to structural damage from blasting is very important for assessment of vibration limits to cover plastic soil deformation effects triggered by blasting. Low Attenuation of Propagated Waves in Saturated Soils C ase 1. Siskind and Stagg (2000) have made a comprehensive study of structural vibration damage to the ten typical houses from quarry blasting. The houses were selected at five areas in Miami-Dade County. Vibration measurements of ground and house structure vibrations were made from 42 quarry blasts during about a 2-month period from late February to late April 2000. The maximum distances between the active quarries and the test sites were in the 3.2-4.8 km (2-3 mi) range. Pre- and post-blasting condition surveys were made before and after vibration monitoring. Additional 756 vibration records from other reports were considered in the study. The soil at the area consisted of 0.3-0.9 m (1-3 ft) of fine grained to fibrous peat followed by 0.6-1.2 m (2-4 ft) of sand followed 0.6 m (2 ft) of marl underlain by weakly to well cemented limestone. The groundwater table is virtually coincidentwith the surface of peat. The specific condition of wave propagation has been found FORENSIC ENGINEERING 2009341D o w n l o a d e d f r o m a s c e l i b r a r y .o r g b y O c e a n U n i v e r s i t y o f C h i n a o n 11/19/13. C o p y r i g h t A S CE .F o r p e r s o n a l u s e o n l y ; a l l r i g h t s r e s e r v e d .in the Miami-Dade County: attenuation with distances is less than found on similar sites in the north. The report has revealed other interesting findings: (a) structure oscillations are superposition of vibrations with various frequencies including low frequencies of about 8 Hz, which are close to the natural frequencies of house horizontal vibrations, and very low frequencies of 2 to 4 Hz; (b) vibrations are of long duration at the houses with maximum duration about 17 second; (c) ground vibrations with the PPV of 19 mm/s (0.75 in./s) and with structure response of 6.1x would produce a global wall strain that is 1.5 times higher of the strain limit for crack formation. On the basis of vibration monitoring in the west and south-west Miami area, the authors concluded that the highest vibrations there would likely be under 3 mm/s (0.12 in/s). Liquefaction in Sands C ase 2. Sanders (1982) has performed an assessment of liquefaction danger from explosively creating breaches in the Bird’s Point – New Madrid fuze plug levee. Creation of two large crevasses would use tens tons of explosives that would cause liquefaction hazards to structures in the area. A rock layer was located at the depth greater than 30 m (100 ft). There were problems with the scarcity of borehole data below 4.5 m (15 ft) and the scarcity of data bearing on the investigation of blast-induced liquefaction. A data base from row charge experiments in soil was used in the assessment of the liquefaction hazards in the area. This study had to determine a possible liquefaction PPV threshold, the maximum distance from a blast where the threshold could be expected, and availability of structures within the distance. The safety criterion of 10 cm/s (3.9 in/s) was found out as the conservative threshold for assessment of liquefaction hazards. The maximum distances at which liquefaction might occur from blasting were ascertained in the 300-383 m (1000-1275 ft) range. The report was reviewed by Dr. H.B. Seed, one of the founders of Earthquake Engineering, who used a totally different line of approach and concluded that the threshold of 10 cm/s (3.9 in/s) was reasonable. Settlement in Sand and Clay Soils C ase 3. Charlie et al. (2001) have reported the potential responses of earth-fill and tailing dams, and other saturated earthen structures subjected to blast vibrations. Eight explosive tests were conducted on a 2.25 m (7.4 ft) high dam constructed of loose dilative sand, and they showed that significant increases in a residual pore pressure occurred when the PPV exceeded 1.5 cm/s (0.59 in/s) at shallow depths to 3.5 cm/s (1.38 in/s) at greater depths. Limited crest settlement occurred when the PPV exceeded 2.5 cm/s (1.0 in/s). Results of research studies and investigation of the field behavior of full-scale earth-fill and tailing dams indicate that the PPV below 2.5 cm/s (0.98 in/s) and 10 cm/s (3.94 in/s) are reasonable thresholds to limit pore pressurebuildup in these dams sensitive and not sensitive to vibrations, respectively.FORENSIC ENGINEERING 2009342D o w n l o a d e d f r o m a s c e l i b r a r y .o r g b y O c e a n U n i v e r s i t y o f C h i n a o n 11/19/13. C o p y r i g h t A S C E . F o r p e r s o n a l u s e o n l y ; a l l r i g h t s r e s e r v e d .C ase 4. Edwards and Northwood (1960) have presented the results of controlled blasting tests of six buildings on two different sites. Damage was correlated with explosive weight, distance, PPV of ground or structure vibrations, settlement and strain measurements in the buildings. The selected buildings were old but in good conditions except for quite localized areas in one or two of them. All had basements constructed with heavy stone masonry walls and frames with brick superstructures. The soil at the first site consisted of 6.1 m (20 ft) loose wet sand underlain by soft marine clay. The water table was about 2.1 m (7 ft) below grade. Sand soil was frozen to a depth of about 0.3 m (1 ft) at the time of the test. The buildings at the first site: church, school and house with 305 mm (12 in) brick walls and plaster inside. Large low-frequency (2-5 Hz) ground vibrations in the sand-clay soils were obtained within hundred feet of the source, and they did not occur at all at 457 m (1,500 ft) and beyond. Damage inspected in the buildings on sand-clay deposits: cracks were mainly vertical and some diagonal cracks developed in the basement and upper walls; cracks were from hairline to 13 mm (0.5 in) width; larger cracks were in walls closest to the blast. The authors concluded that the damage in the buildings on sand-clay deposits was caused by failure of the soil manifested in settlements under the building rather than by vibrations of building structures. The threshold level of 102-127 mm (4-5 in/s) was defined. Damage to Foundation Walls C ase 5. Edwards and Northwood (1960) have conducted experiments of controlled blasting operations near buildings with the objective of producing damage. General information is the same as in Case 4. The soil at the second site comprised well-consolidated glacial till which is a high shear strength material consisting of a highly compacted mixture of sand, clay, gravel and boulders. Till was frozen to a depth of about 1 ft at the time of the test. There were three houses at the second site: two with 305 mm (12 in) brick walls and plaster inside, and third with frame and vinyl sliding and wood fiber board inside. Most of the high-frequency components of ground vibrations were obtained in the till soils. Damage inspected in the buildings on till: some horizontal cracks; most of the windows in the ground floor longitudinal walls were broken while those in the walls normal to the blast remained intact. Major damage was inflicted on one building on till soil. Considerable section of the rear basement wall fell away and the masonry walls above ground level were bulged out approximately 76 mm (3 in). The threshold level of 102-127 mm (4-5 in/s) was defined. Vibration Limits and Surveys of StructuresCase histories presented above demonstrate various soil contributions to blasting effects on wave propagation and soil failure. Wave paths in saturated soils underlain FORENSIC ENGINEERING 2009343D o w n l o a d e d f r o m a s c e l i b r a r y .o r g b y O c e a n U n i v e r s i t y o f C h i n a o n 11/19/13. C o p y r i g h t A S CE .F o r p e r s o n a l u s e o n l y ; a l l r i g h t s r e s e r v e d .by rock had low attenuation and as a result ground vibrations had higher PPV. Consequently, the vibration limit of ground vibrations was decreased to 3 mm/s (0.12 in/s). This criterion is four times less than the smallest USBM vibration limit. However, the vibration thresholds of soil failure like liquefaction, settlement and soil movement damaged foundation walls were 1.5-5 times higher then the highest USBM limit in most cases.The obtained results were determined for the specific cases, and the application of these criteria to other sites can be used only for preliminary assessment of blasting effects because each site is unique. It is necessary to keep in mind that changes of blast parameters, wave paths and structure types may yield different outcomes and require different safe criteria, Siskind et al. (1980), Siskind (1991), Siskind and Stagg (2000), Svinkin (2006, 2007). Such criteria are not always available. Therefore in addition to vibration measurements, it is imperative to use other approaches like the condition survey of houses and buildings that provides good opportunities for assessment of blasting damage to structures.Pre- and post-blast surveys of structures have to be professionally performed because different kind of static and dynamic loading like environmental forces, geotechnical hazards, and dynamic effects of blasting can be the causes of similar structural damage, and such damage can exist before the beginning of blasting. It is common to consider only the effects of environmental forces such as temperature, humidity, wind, rain, snow, etc. on structural integrity. However, it is particularly important to analyze the geotechnical natural hazards at various distances from construction sites such as heave, settlement, stability, sliding and failure which may damage structures prior to construction, during and after construction. There are case histories which show the major effects of environmental forces and geotechnical causes on crack formation and structural damage in spite of blasting at various distances from houses and buildings. For example, the writer analyzed the results of pre- and post-blast structure surveys of the building and found that the geotechnical hazard, a soil failure started prior to the beginning of blasting, was the cause of damage to structures.A pre-construction survey is the first step in the control of structural vibrations to ensure safety and serviceability of adjacent and remote houses, buildings and facilities. There are four goals of this survey: (1) document the existing cracks and other damage; (2) ascertain the cause(s) of the existing cracks and other damage; (3) assess susceptibility rating of structures; (4) determine mitigation measures of blasting effects on structures.At some sites, the condition survey can provide more information about benign and harmful blasting effects on structures than vibration measurements performed during blast operations because the vibration criteria cannot embrace the whole spectrum of the blast effects on structures. The condition surveys must always accompany measurements of blasting vibrations. Surveys of structures and analysis of damage to structures should be performed by engineers who have an experience in Soil-Structural Dynamics and evaluation of blast effects on soil and structures. For FORENSIC ENGINEERING 2009344D o w n l o a d e d f r o m a s c e l i b r a r y .o r g b y O c e a n U n i v e r s i t y o f C h i n a o n 11/19/13. C o p y r i g h t A S C E . F o r p e r s o n a l u s e o n l y ; a l l r i g h t s r e s e r v e d .。