Hybrid_pneumatic_ICE_2011
谷轮ZW系列(中间补气涡旋)压缩机应用指南

AE4-1381May 2011ZW21 to ZW61KAE and ZW30 to ZW61KSECopeland Scroll® Water Heating CompressorsTABLE OF CONTENTSSection Page Section PageIntroduction (2)ZW**KA Application (2)ZW**KS ApplicationVapour Injection - Theory of Operation (2)Heat Exchanger and Expansion Device Sizing (3)Flash Tank Application (3)Intermediate Pressure and Vapour Injection Superheat (3)Application ConsiderationsHigh Pressure Cut-Out (4)Low Pressure Cut-Out (4)Discharge Temperature Protection (4)Discharge Temperature Control (4)Discharge Mufflers (4)Oil Dilution and Compressor Cooling (4)Electrical Considerations (5)Brazing and Vapour Injection Line (5)Low Ambient Cut-Out (5)Internal Pressure Relief Valve (5)Internal Temperature Protection (5)Quiet Shutdown (5)Discharge Check Valve (5)Motor Protector (5)Accumulators (5)Screens (6)Crankcase Heat-Single Phase (6)Crankcase Heat-Three Phase (6)Pump Down Cycle (6)Minimum Run Time (6)Reversing Valves (6)Oil Type (7)System Noise & Vibration (7)Single Phase Starting Characteristics (7)PTC Start Components (7)Electrical Connections (7)Deep Vacuum Operation (7)Shell Temperature (7)Suction & Discharge Fittings (7)Three Phase Scroll Compressors (8)Brief Power Interruptions ..........................................8Assembly Line ProceduresInstalling the Compressor (8)Assembly Line Brazing Procedure (8)Pressure Testing (8)Assembly Line System Charging Procedure (8)High Potential (AC Hipot) Testing (9)Unbrazing System Components (9)Service ProceduresCopeland Scroll Functional Check (9)Compressor Replacement After Motor Burn (10)Start Up of a New or Replacement Compressor (10)FiguresBrief Product Overview (11)ZW21KAE Envelope (R-134a) (11)ZWKAE Envelope (R-407C, Dew Point) (12)ZWKA Envelope (R-22) (12)ZWKS Envelope (R-22) (13)ZWKSE Envelope (R-407C, Dew Point) (13)Heat Pump with Vapour Injection – EXV Control (14)Heat Exchanger Schematic (14)Heat Pump with Flash Tank (15)Possible Flash Tank Configuration (15)Oil Dilution Chart (16)Crankcase Heater (17)Compressor Electrical Connection (17)Scroll Tube Brazing (17)How a Scroll Works (18)IntroductionThe ZW**KA and ZW**KS Copeland Scroll®compressors are designed for use in vapour compression heat pump water heating applications. Typical model numbers include ZW30KA-PFS and ZW61KSE-TFP. This bulletin addresses the specifics of water heating in the early part and deals with the common characteristics and general application guidelines for Copeland Scroll compressors in the later sections. Operating principles of the scroll compressor are described in Figure 15 at the end of this bulletin.As the drive for energy efficiency intensifies, water heating by fossil-fueled boilers and electric elements is being displaced by vapour compression heat pumps. Emerson Climate Technologies has developed two lines of special water heater compressors to meet the requirements of this demanding application. ZW**KA compressors are designed for lighter duty applications where the ambient temperature does not fall below 0°C and where lower water temperatures can be accepted as the ambient temperature falls. ZW**KS compressors are equipped with a vapour injection cycle which allows reliable operation in cold climates with significantly enhanced heating capacity, higher efficiency, and minimal requirement to reduce water outlet temperatures. Figure 1gives a brief product overview.Water heating is characterized by long operating hours at both high load and high compression ratios. Demand for hot water is at its highest when ambients are low and when conventional heat pump capacity falls off. On the positive side, the system refrigerant charge is usually small, so the risk to the compressor from dilution and flooded starts will usually be lower than in split type air-to-air heat pumps.Water heaters must operate in a wide range of ambient temperatures, and many systems will require some method of defrost. Some systems such as Direct Heating, Top Down Heating or Single Pass Heating operate at a constant water outlet temperature with variable water flow. Others such as Recirculation Heating, Cyclic Heating or Multipass Heating use constant water flow with the water outlet and inlet temperatures both rising slowly as the storage tank heats up. Both system types need to cope with reheating a tank where the hot water has been partially used, and reheating to the setpoint temperature is required. More complex systems deliver water at relatively low temperatures for under-floor heating circuits and are switched over to sanitary water heating a few times per day to provide higher temperature water for sanitary use. In addition, some countries have specific water temperature requirements for legionella control.ZW**KA ApplicationThe application envelopes for ZW**KA compressors are shown in Figures 2 - 4.Appropriate system hardware and control logic must be employed to ensure that the compressor is always operating within the envelope. Small short-term excursions outside the envelope are acceptable at the time of defrost when the load on the compressor is low. Operation with suction superheat of 5 -10K is generally acceptable except at an evaporating tem-perature above 100C when a minimum superheat of 10K is required.ZW**KS ApplicationThe ZW**KS* vapour-injected scroll compressors differ from ZW**KA models in many important details:• Addition of vapour injection• Significantly different application envelopes• Some differences in locked rotor amps (LRA), maximum continuous current (MCC), andmaximum operating current (MOC) – seenameplatesThe application envelopes for ZW**KS compressors are shown in Figures 5 and 6.Vapour Injection – Theory of Operation Operation with vapour injection increases the capacity of the outdoor coil and in turn the capacity and efficiency of the system – especially in low ambient temperatures. A typical schematic is shown in Figure 7. A heat exchanger is added to the liquid line and is used to cool the liquid being delivered to the heating expansion device. Part of the liquid refrigerant flow is flashed through an expansion valve on the evaporator side of the heat exchanger at an intermediate pressure and used to subcool the main flow of liquid to the main expansion device. Vapour from the liquid evaporating at intermediate pressure is fed to the vapour injection port on the ZW**KS compressor. This refrigerant is injected into the mid-compression cycle of the scroll compressor and compressed to discharge pressure. Heating capacity is increased, because low temperature liquid with lower specific enthalpy supplied to the outdoor coil increases the amount of heat that can be absorbed from the ambient air. Increased heat absorbed from the ambient increases the system condensing temperature and in turn the compressor power input. The increase in power inputalso contributes to the improvement in the overall heating capacity.Vapour Injection can be turned on and off by the addition of an optional solenoid valve on the vapour injection line on systems using a thermostatic expansion valve. Alternatively, an electronic expansion valve can be used to turn vapour injection on and off and to control the vapour injection superheat. A capillary tube is not suitable for controlling vapour injection.The major advantage of the electronic expansion valve is that it can be used to optimise the performance of the system and at the same time control the discharge temperature by injecting “wet vapour” at extreme operating conditions.The configurations and schematics shown are for reference only and are not applicable to every system. Please consult with your Emerson Application Engineer.Heat Exchanger and Expansion Device Sizing Various heat exchanger designs have been used successfully as subcoolers. In general they should be sized so that the liquid outlet temperature is less than 5K above the saturated injection temperature at the customer low temperature rating point. At very high ambient temperatures, it will normally be beneficial to turn vapour injection off to limit the load on the compressor motor. Application Engineering Bulletin AE4-1327 and Emerson Climate Technologies Product Selection Software can be used to help size the subcooling heat exchanger and thermal expansion valves, but selection and proper operation must be checked during development testing. Plate type subcoolers must be installed vertically with the injection expansion device connected at the bottom through a straight tube at least 150mm long to ensure good liquid distribution. See the schematic in Figure 8. Flash Tank ApplicationA possible flash tank configuration is shown in Figure9. This particular configuration is arranged to have flow through the flash tank and expansion devices in heating, and it bypasses the tank in defrost mode. The flash tank system works by taking liquid from the condenser and metering it into a vessel through a high-to-medium pressure expansion device. Part of the liquid boils off and is directed to the compressor vapour injection port. This refrigerant is injected into the mid-compression cycle of the scroll compressor and compressed to discharge pressure. The remaining liquid is cooled, exits from the bottom of the tank at intermediate pressure, and flows to the medium-to-low pressure expansion device which feeds the outdoor coil. Low temperature liquid with lower specific enthalpy increases the capacity of the evaporator without increasing mass flow and system pressure drops.Recommended tank sizing for single compressor application in this size range is a minimum of 200 mm high by 75 mm in diameter with 3/8 in. (9.5mm) tubing connections, although it is possible to use a larger tank to combine the liquid/vapour separation and receiver functions in one vessel. A sight tube (liquid level gauge) should be added to the tank for observation of liquid levels during lab testing. See schematic diagram Figure 10 for clarification.It is important to maintain a visible liquid refrigerant level in the tank under all operating conditions. Ideally the liquid level should be maintained in the 1/3 to 2/3 full range.Under no circumstances should the level drop to empty or rise to a full tank. As the tank level rises, liquid droplets tend to be swept into the vapour line leading to “wet” vapour injection. Although this can be useful for cooling a hot compressor, the liquid quantity cannot be easily controlled. Compressor damage is possible if the tank overflows. If liquid injection is required for any reason, it can be arranged as shown in Figures 7 and 9.Since liquid leaves the tank in a saturated state, any pressure drop or temperature rise in the line to the medium-to-low pressure expansion device will lead to bubble formation. Design or selection of the medium-to-low pressure expansion device requires careful attention due to the possible presence of bubbles at the inlet and the low pressure difference available to drive the liquid into the evaporator. An electronic expansion valve is the preferred choice. Intermediate Pressure and Vapour Injection SuperheatPressure in the flash tank cannot be set and is a complex function of the compressor inlet condition and liquid condition at the inlet of the high-to-medium pressure expansion device. However, liquid level can be adjusted, which in turn will vary the amount of liquid subcooling in the condenser (water to refrigerant heat exchanger) and vary the injection pressure. Systems with low condenser subcooling will derive the biggest gains by the addition of vapour injection. Systems operating with high pressure ratios will show the largest gains when vapour injection is applied. Such systems will have higher vapour pressure and higher injectionmass flow. Intermediate pressures in flash tank and heat exchanger systems should be very similar unless the subcooling heat exchanger is undersized and there is a large temperature difference between the evaporator and the liquid sides. Vapour exiting a flash tank will be saturated and may pick up 1 - 2K superheat in the vapour line to the compressor. Vapour injection superheat cannot be adjusted on flash tank systems. Heat exchanger systems will be at their most efficient when the vapour injection superheat is maintained at approximately 5K.APPLICATION CONSIDERATIONSHigh Pressure Cut OutIf a high pressure control is used with these compressors, the recommended maximum cut out settings are listed in Figure 1. The high pressure control should have a manual reset feature for the highest level of system protection. It is not recommended to use the compressor to test the high pressure switch function during the assembly line test.Although R-407C runs with higher discharge pressure than R-22, a common setting can be used. The cutout settings for R-134a are much lower, and the switches must be selected or adjusted accordingly.Low Pressure Cut OutA low pressure cut out is an effective protection against loss of charge or partial blockage in the system. The cut out should not be set more than 3 - 5K equivalent suction pressure below the lowest operating point in the application envelope. Nuisance trips during defrost can be avoided by ignoring the switch until defrost is finished or by locating it in the line between the evaporator outlet and the reversing valve. This line will be at discharge pressure during defrost. Recommended settings are given in Figure 1. Discharge Temperature ProtectionAlthough ZW compressors have an internal bi-metal Therm-O-Disc®(TOD) on the muffler plate, external discharge temperature protection is recommended for a higher level of protection and to enable monitoring and control of vapour injection on ZW**KS* models. The protection system should shut down the compressor when the discharge line temperature reaches 125°C. In low ambient operation, the temperature difference between the scroll center and the discharge line is significantly increased, so protection at a lower discharge temperature, e.g. 120°C when the ambient is below 0°C, will enhance system safety. For the highest level of system protection, the discharge temperature control should have a manual reset feature. The discharge sensor needs to be well insulated to ensure that the line temperature is accurately read. The insulation material must not deteriorate over the expected life of the unit.Discharge Temperature ControlSome systems use an electronic expansion valve to control the vapour injection superheat and a thermistor to monitor the discharge temperature. This combination allows the system designer to inject a small quantity of liquid to keep the discharge temperature within safe limits and avoid an unnecessary trip. Liquid injection should begin at approximately 115°C and should be discontinued when the temperature falls to 105°C. Correct functioning of this system should be verified during system development. It is far preferable to use liquid injection into the vapour injection port to keep the compressor cool rather than inject liquid into the compressor suction which runs the risk of diluting the oil and washing the oil from the moving parts. If some operation mode requires liquid injection but without the added capacity associated with “wet” vapour injection, a liquid injection bypass circuit can be arranged as shown in Figures 7 and 9.Caution: Although the discharge and oil temperature are within acceptable limits, the suction and discharge pressures cannot be ignored and must also fall within the approved application envelope.Discharge MufflersDischarge mufflers are not normally required in water heaters since the refrigerant does not circulate within the occupied space.Oil Dilution and Compressor CoolingThe oil temperature diagram shown in Figure 11is commonly used to make a judgment about acceptable levels of floodback in heat pump operation. Systems operating with oil temperatures near the lower limit line are never at their most efficient. Low ambient heating capacity and efficiency will both be higher if floodback is eliminated and the system runs with 1 - 5K suction superheat. Discharge temperature can be controlled by vapour injection, “wet” vapour injection, or even liquid injection if necessary. In this situation, the oil temperature will rise well into the safe zone, and the compressor will not be at risk of failure from diluted oil. The oil circulation rate will also be reduced as crankcase foaming disappears. Special care needs to be taken at the end of defrost to ensure that the compressor oil is not unacceptably diluted. The system will resume heating very quickly and bearing loads willincrease accordingly, so proper lubrication must be ensured.Electrical ConsiderationsMotor configuration and protection are similar to those of standard Copeland Scroll compressors. In some cases, a larger motor is required in the ZW**KS* models to handle the load imposed by operating with vapour injection. Wiring and fuse sizes should be reviewed accordingly.Brazing the Vapour Injection LineThe vapour injection connection is made from copper coated steel, and the techniques used for brazing the suction and discharge fittings apply to this fitting also. Low Ambient Cut-OutA low ambient cut-out is not required to limit heat pump operation with ZW**KS compressors. Water heaters using ZW**KA compressors must not be allowed to run in low ambients since this configuration would run outside of the approved operating envelope causing overheating or excessive wear. A low ambient cut-out should be set at 0°C for ZW**KA modelsIn common with many Copeland Scroll compressors, ZW models include the features described below: Internal Pressure Relief (IPR) ValveAll ZW compressors contain an internal pressure relief valve that is located between the high side and the low side of the compressor. It is designed to open when the discharge-to-suction differential pressure exceeds 26 - 32 bar. When the valve opens, hot discharge gas is routed back into the area of the motor protector to cause a trip.Internal Temperature ProtectionThe Therm-O-Disc® or TOD is a temperature-sensitive snap disc device located on the muffler plate between the high and low pressure sides of the compressor. It is designed to open and route excessively hot discharge gas back to the motor protector. During a situation such as loss of charge, the compressor will be protected for some time while it trips the protector. However, as refrigerant leaks out, the mass flow and the amperage draw are reduced and the scrolls will start to overheat.A low pressure control is recommended for loss of charge protection in heat pumps for the highest level of system protection. A cut out setting no lower than 2.5 bar for ZW**KA* models and 0.5 bar for ZW**KS* models is recommended. The low pressure cut-out, if installed in the suction line to the compressor, can provide additional protection against an expansion device failed in the closed position, a closed liquid line or suction line service valve, or a blocked liquid line screen, filter, orifice, or TXV. All of these can starve the compressor for refrigerant and result in compressor failure. The low pressure cut-out should have a manual reset feature for the highest level of system protection. If a compressor is allowed to cycle after a fault is detected, there is a high probability that the compressor will be damaged and the system contaminated with debris from the failed compressor and decomposed oil.If current monitoring to the compressor is available, the system controller can take advantage of the compressor TOD and internal protector operation. The controller can lock out the compressor if current draw is not coincident with the contactor energizing, implying that the compressor has shut off on its internal protector. This will prevent unnecessary compressor cycling on a fault condition until corrective action can be taken.Quiet Shut downAll scrolls in this size range have a fast acting valve in the center of the fixed scroll which provides a very quiet shutdown solution. Pressure will equalize internally very rapidly and a time delay is not required for any of the ZW compressors to restart. Also refer to the section on “Brief Power Interruption”. Discharge Check ValveA low mass, disc-type check valve in the discharge fitting of the compressor prevents the high side, high pressure discharge gas from flowing rapidly back through the compressor. This check valve was not designed to be used with recycling pump down because it is not entirely leak-proof.Motor ProtectorConventional internal line break motor protection is provided. The protector opens the common connection of a single-phase motor and the center of the Y connection on three-phase motors. The three-phase protector provides primary single-phase protection. Both types of protectors react to current and motor winding temperature.AccumulatorsThe use of accumulators is very dependent on the application. The scroll’s inherent ability to handle liquid refrigerant during occasional operating flood back situations often makes the use of an accumulator unnecessary in many designs. If flood back is excessive, it can dilute the oil to such an extent thatbearings are inadequately lubricated, and wear will occur. In such a case, an accumulator must be used to reduce flood back to a safe level that the compressor can handle.In water heaters, floodback is likely to occur when the outdoor coil frosts. The defrost test must be done at an outdoor ambient temperature of around 0°C in a high humidity environment. Liquid floodback must be monitored during reversing valve operation, especially when coming out of defrost. Excessive floodback occurs when the sump temperature drops below the safe operation line shown in Figure 11 for more than 10 seconds.If an accumulator is required, the oil return orifice should be 1 - 1.5mm in diameter depending on compressor size and compressor flood back results. Final oil return hole size should be determined through testing. ScreensScreens with a mesh size finer than 30 x 30 (0.6mm openings) should not be used anywhere in the system with these compressors. Field experience has shown that finer mesh screens used to protect thermal expansion valves, capillary tubes, or accumulators can become temporarily or permanently plugged with normal system debris and block the flow of either oil or refrigerant to the compressor. Such blockage can result in compressor failure.Crankcase Heater - Single PhaseCrankcase heaters are not required on single phase compressors when the system charge is not over 120% of the limit shown in Figure 1. A crankcase heater is required for systems containing more than 120% of the compressor refrigerant charge limit listed in Figure 1. This includes long line length systems where the extra charge will increase the standard factory charge above the 120% limit.Experience has shown that compressors may fill with liquid refrigerant under certain circumstances and system configurations, notably after longer off cycles when the compressor has cooled. This may cause excessive start-up clearing noise, or the compressor may lock up and trip on the protector several times before starting. The addition of a crankcase heater will reduce customer noise and light dimming complaints since the compressor will no longer have to clear out liquid during startup. Figure 12lists the crankcase heaters recommended for the various models and voltages.Crankcase Heat – Three-PhaseA crankcase heater is required for three-phase compressors when the system charge exceeds the compressor charge limit listed in Figure 1and an accumulator cannot be piped to provide free liquid drainage during the off cycle.Pump Down CycleA pump down cycle for control of refrigerant migration is not recommended for scroll compressors of this size. If a pump down cycle is used, a separate external check valve must be added.The scroll discharge check valve is designed to stop extended reverse rotation and prevent high-pressure gas from leaking rapidly into the low side after shut off. The check valve will in some cases leak more than reciprocating compressor discharge reeds, normally used with pump down, causing the scroll compressor to cycle more frequently. Repeated short-cycling of this nature can result in a low oil situation and consequent damage to the compressor. The low-pressure control differential has to be reviewed since a relatively large volume of gas will re-expand from the high side of the compressor into the low side on shut down. Minimum Run TimeThere is no set answer to how often scroll compressors can be started and stopped in an hour, since it is highly dependent on system configuration. Other than the considerations in the section on Brief Power Interruptions, there is no minimum off time. This is because scroll compressors start unloaded, even if the system has unbalanced pressures. The most critical consideration is the minimum run time required to return oil to the compressor after startup.Since water heaters are generally of compact construction, oil return and short cycling issues are rare. Oil return should not be a problem unless the accumulator oil hole is blocked.Reversing ValvesSince Copeland Scroll compressors have very high volumetric efficiency, their displacements are lower than those of comparable capacity reciprocating compressors. As a result, Emerson recommends that the capacity rating on reversing valves be no more than 2 times the nominal capacity of the compressor with which it will be used in order to ensure proper operation of the reversing valve under all operating conditions.The reversing valve solenoid should be wired so that the valve does not reverse when the system isshut off by the operating thermostat in the heating or cooling mode. If the valve is allowed to reverse at system shutoff, suction and discharge pressures are reversed to the compressor. This results in pressures equalizing through the compressor which can cause the compressor to slowly rotate until the pressures equalize. This condition does not affect compressor durability but can cause unexpected sound after the compressor is turned off.Oil TypeThe ZW**K* compressors are originally charged with mineral oil. A standard 3GS oil may be used if the addition of oil in the field is required. See the compressor nameplate for original oil charge. A complete recharge should be ~100 ml less than the nameplate value.ZW**K*E are charged with POE oil. Copeland 3MAF or Ultra 22 CC should be used if additional oil is needed in the field. Mobil Arctic EAL22CC, Emkarate RL22, Emkarate 32CF and Emkarate 3MAF are acceptable alternatives. POE oil is highly hygroscopic, and the oil should not be exposed to the atmosphere except for the very short period required to make the brazing connections to the compressor.System Noise and VibrationCopeland Scroll compressors inherently have low sound and vibration characteristics, but the characteristics differ in some respects from those of reciprocating or rotary compressors. The scroll compressor makes both a rocking and a torsional motion, and enough flexibility must be provided to prevent vibration transmission into any lines attached to the unit. This is usually achieved by having tubing runs at least 30cm long parallel to the compressor crankshaft and close to the shell. ZW compressors are delivered with rubber grommets to reduce vibration transmission to the system baseplate.Single Phase Starting CharacteristicsStart assist devices are usually not required, even if a system utilizes non-bleed expansion valves. Due to the inherent design of the Copeland Scroll, the internal compression components always start unloaded even if system pressures are not balanced. In addition, since internal compressor pressures are always balanced at startup, low voltage starting characteristics are excellent for Copeland Scroll compressors. Starting current on any compressor may result in a significant “sag” in voltage where a poor power supply is encountered. The low starting voltage reduces the starting torque of the compressor and subsequently increases the start time. This could cause light dimming or a buzzing noise where wire is pulled through conduit. If required, a start capacitor and potential relay can be added to the electrical circuit. This will substantially reduce start time and consequently the magnitude and duration of both light dimming and conduit buzzing.PTC Start ComponentsFor less severe voltage drops or as a start boost, solid state Positive Temperature Coefficient devices rated from 10 to 25 ohms may be used to facilitate starting for any of these compressors.Electrical ConnectionThe orientation of the electrical connections on the Copeland Scroll compressors is shown in Figure 13 and is also shown on the wiring diagram on the top of the terminal box cover.Deep Vacuum OperationScrolls incorporate internal low vacuum protection and will stop pumping (unload) when the pressure ratio exceeds approximately 10:1. There is an audible increase in sound when the scrolls start unloading. This feature does not prevent overheating and destruction of the scrolls, but it does protect the power terminals from internal arcing.Copeland Scroll compressors(as with any refrigerant compressor) should never be used to evacuate a refrigeration or air conditioning system. The scroll compressor can be used to pump down refrigerant in a unit as long as the pressures remain within the operating envelope. Prolonged operation at low suction pressures will result in overheating of the scrolls and permanent damage to the scroll tips, drive bearings and internal seal. (See AE24-1105 for proper system evacuation procedures.)Shell TemperatureCertain types of system failures, such as condenser or evaporator blockage or loss of charge, may cause the top shell and discharge line to briefly but repeatedly reach temperatures above 175ºC as the compressor cycles on its internal protection devices. Care must be taken to ensure that wiring or other materials, which could be damaged by these temperatures, do not come in contact with these potentially hot areas. Suction and Discharge FittingsCopeland Scroll compressors have copper plated steel suction and discharge fittings. These fittings are far more rugged and less prone to leaks than。
约克空调操作手册
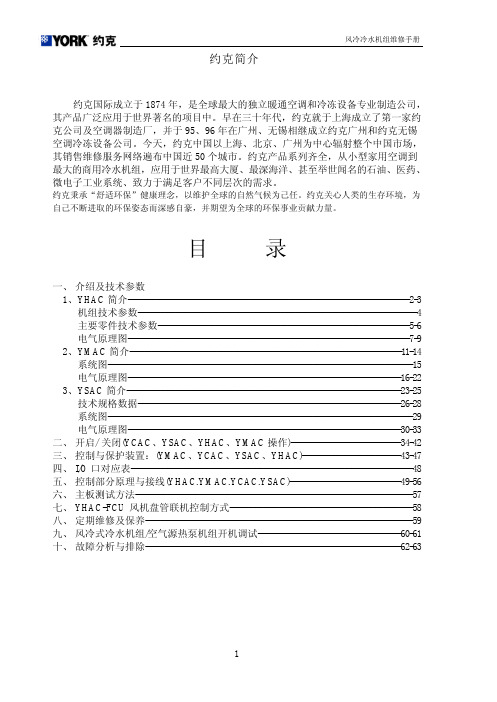
2
风冷冷水机组维修手册
注:YHAC50C,YHAC50H,YHAC60C,YHAC60H, YHAC80C,YHAC80H 外形图。
注:YHAC40C,YHAC40H , YHAC33C,YHAC33H, YHAC25C,YHAC25H, YHAC20C,YHAC20H 外形图
3
4、机组技术参数:
缩 连接方式 机 能力调节
∆
0,100%
∆
0,100%
∆
0,100%
∆
0,100%
∆
0,100%
∆
0,100%
润滑油量类型
白油 Sontex 200LT (现场可替代:3GS) SUNISO4GSDID-K 160P
润滑油量 L 油加热功率(W) 电源
3.48 40
4.43 40
制冷量(kW) 制热量(kW)
辅助制热量(kW) 辅助制热量(kW) 辅助制热量(kW)
YHAC20H(P)
18
21
6
4
3
YHAC25H(P)
24
26
8
6
4
YHAC 系列 YHAC40H(P)
40
43
14
9
7
YHAC50H
50
54
18
12
9
YHAC60H
60
YHAC60H 60 65
6.4*2 8.0*2 12.3*2 12.3*2
17
8600
17
22
11180
21
35
127
17
35
42
phoenics2011新功能
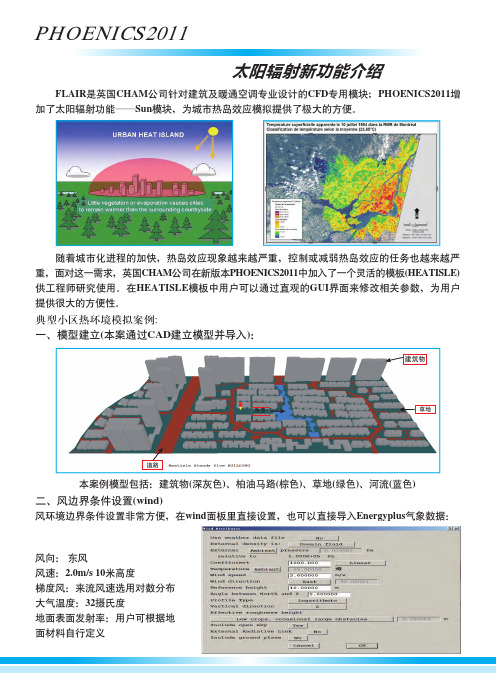
随着城市化进程的加快,热岛效应现象越来越严重,控制或减弱热岛效应的任务也越来越严重,面对这一需求,英国CHAM公司在新版本PHOENICS2011中加入了一个灵活的模板(HEATISLE)供工程师研究使用。
在HEATISLE模板中用户可以通过直观的GUI界面来修改相关参数,为用户提供很大的方便性。
典型小区热环境模拟案例:
一、模型建立(本案通过CAD建立模型并导入
水系建筑物
草地
道路
六、结果:阴影、日照强度赋值情况、表面温度等
四、其他边界条件的设置:天空辐射、建筑物、草地、道路、土壤等的属性都可以在相应 的object 属性里详细设置。
三、热环境边界的设置(SUN)
热环境边界条件设置可在Sun 的
属性面板里直接设置:
当地维度:51
太阳直射辐射强度:500
太阳散射辐射强度:100
模拟时间:2011年7月1日12时
同样也可直接导入Energyplus
气象数据:
五、网格自动生成
蓝色箭头为指北针,红色箭头为风向标
(本案例风向为东风)太阳的位置:红色大球为太阳的位置(12时),其他小球为太阳的小时位置图
建筑表面温度图建筑阴影图建筑、路面等表面日照强度赋值情况图。
- 1、下载文档前请自行甄别文档内容的完整性,平台不提供额外的编辑、内容补充、找答案等附加服务。
- 2、"仅部分预览"的文档,不可在线预览部分如存在完整性等问题,可反馈申请退款(可完整预览的文档不适用该条件!)。
- 3、如文档侵犯您的权益,请联系客服反馈,我们会尽快为您处理(人工客服工作时间:9:00-18:30)。
Type Paper Number Here One Dimensional Modeling and Experimental Validation of SingleCylinder Pneumatic Combustion Hybrid Engine Pascal BREJAUD, Pascal HIGELIN, Alain CHARLET, Yann CHAMAILLARD (Do NOT enter this information. It will be pulled from participant tab in MyTechZone)Affiliation (Do NOT enter this information. It will be pulled from participant tab in MyTechZone) Copyright © 2011 SAE InternationalABSTRACTThe objective of this paper is to present and to validate a numerical model of a single-cylinder pneumatic-combustion hybrid engine. The model presented in this paper contains 0-D sub-models for non-spatially distributed components: Engine cylinder, Air tank, wall heat losses. 1-D sub-models for spatially distributed components are applied on the compressive gas flows in pipes (intake, exhaust and charging). Each pipe is discretized, using the Two-Steps Lax-Wendroff scheme (LW2) including Davis T.V.D. The boundaries conditions used at pipe ends are Method Of Characteristics (MOC) based. In the specific case of a valve, an original intermediate volume MOC based boundary condition is used. The numerical results provided by the engine model are compared with the experimental data obtained from a single cylinder prototype hybrid engine on a test bench operating in 4-stroke pneumatic pump and 4 stroke pneumatic motor modes. In each mode, the prediction of the mass flow rates, amplitude and timing of the charging pipe waves are satisfactory, without using any discharge coefficient. Indicated work and p-V diagrams are similar between simulation and measurements in the case of pneumatic pump mode. For the pneumatic motor mode the model underestimates cylinder pressure during the charging process.INTRODUCTIONFigure 1: Schematic representation of a Single CylinderThe concept of the pneumatic hybrid engine (1) (2) is based on a conventional internal combustion engine in which one additional valve, called the charging valve, connects the combustion chamber to an air tank. See Figure 1. In order to reduce costs, theintake and exhaust valve remain camshaft-driven and only the charging valve is moved by a fully variable actuator (3). As a result, thenew major modes allowed by this additional valve (pneumatic pump, pneumatic motor and supercharged) remains 4-Strokes cycles:Page 1 of 16Page 2 of 16 ∙Pneumatic Pump : This mode transforms vehicle kinetic energy into a re-usable pneumatic energy. The cycle begins with a conventional intake stroke. The charging valve opens during compression strokes as soon as the in-cylinder pressure reaches that of the charging port. The valve closes shortly after T.D.C, and the cycle finishes with conventional expansion and exhaust strokes.∙Pneumatic Motor : This mode is used to transform pneumatic energy into mechanical work without any combustion. The cycle begins with classical intake and compression strokes. The charging valve opens when approaching TDC, and remains open during part of the expansion stroke in order to maintain pressure at a high level and generate the desired indicated work. The cycle finishes with a conventional exhaust stroke.∙Pneumatic Supercharged mode : This mode is used to compensate for the "turbo lag" effect, while temporarily boost the engine during a strong acceleration transient. The charging valve opens shortly after intake valve closure, and closes when the desired additional air mass has flowed into the in-cylinder. The end of the cycle remains the same as a conventional four stroke one.Several previous publications (4) (5) (6) have shown that the concept of a pneumatic-combustion hybrid engine could provide benefits in terms of fuel consumption and greenhouse gas emissions that are comparable with those of an electric hybrid vehicle (7) but with lower weight and presumably lower cost (6). To improve the performance and efficiency of the hybrid pneumatic concept, the development mainly consists in determining the timing of opening and closing of the charging valve, for a given operating mode (pneumatic motor mode, pneumatic pump mode, supercharged mode) and for a given objective (maximization or nominal control of mechanical work, maximization or nominal control of mass or enthalpy transferred, maximization of global efficiency etc.). It has been experimentally shown (8) that large amplitude pressure waves (near 2 bars of amplitude) are created inside the charging pipe as a result of the charging valve opening and closure. In order to take this pressure wave phenomenon into account a 1D simulation platform is needed.However, the way in which gas transfer between the cylinder and the Air tank is performed depends on many parameters, such as: charging pipe length and cross section, air tank mean pressure, air tank temperature, charging valve hydraulic diameter and number, charging pipe roughness, charging valve lift. The large number of parameters to be explored for each operating mode and each objective makes optimization almost impossible to perform experimentally. A reliable, flexible, and fast numerical 1D model is therefore needed in order to develop control laws. Two previous studies (9) (10) have used a 1-D commercial simulation software for engine simulations, but do not present any comparison between experimental and simulated results for wave timings and amplitude, mass flow rates etc. From our point of view, the use of such commercial code for a pneumatic hybrid engine poses one problem and leaves one question unanswered. The problem is that the software requires a precise knowledge of the discharge coefficient versus lift of each simulated valve (11). These coefficient arrays have to be determined by experiments thus a prototype cylinder head is required. An estimated discharge coefficient will therefore be more or less erroneous, leading to serious discrepancies between simulations and future measurements. The unanswered question is that the valve boundary condition implemented in this kind of software is seldom validated on pressure ratios as high as those encountered in a pneumatic hybrid engine (up to 22). To circumvent the previous points, authors have developed their own MOC valve boundary condition that obviates in many cases the use of a Coefficient of discharge and has been experimentally validated on a valve gas dynamics test bench (12). The objective of the paper is now to present the complete engine model that embeds this valve boundary condition, and to compare experiments versus simulations.The paper is organized as follows: Firstly, 0D components models are described: the cylinder and the air tank model. Secondly, the 1D sub models for spatially distributed components are described: the LW2 numerical scheme and the simplified TVD scheme proposed by Davis are presented, followed by the Intermediate Volume Valve boundary condition. Thirdly the results produced by the complete single cylinder hybrid engine are presented and compared to measurements obtained with a prototype engine.QUASI-DIMENSIONAL COMPONENTS OF THE ENGINE MODEL AIR TANK:The air tank thermodynamic state shown on Figure 1is determined using the first law of thermodynamics:.i dU W Q h dm δδ=++(1)Page 3 of 16 Because the tank has a constant volume, 0WpdV δ=-=. According to Joule's law v v dU mc dT c Tdm =+, with Tthe temperature of the gases contained in the Tank. The air tank is assumed to be adiabatic, then 0Q δ=. For a perfect gas, the specific enthalpy is given by i p i h c T =, with i T the temperature of the flowing gases. Finally, a re-arrangement of Eq. (1) gives:().p i v vc T c T dmdT mc -=(2)The air flow mass dm is determined at the open end boundary (see corresponding section of this paper) using the method of characteristics (M.O.C). A classical numerical integration then provides the total air mass inside the tank. Using Eq. (2), the elementary temperature rise in the tank is determined. Applying a numerical integration, the new tank temperature is computed. Finally, using the perfect gas law (pV=mrT), the mean tank pressure is determined.ENGINE CYLINDERThe cylinder is the central part of the system. It exchanges heat and mass with the outside. Its thermodynamic state is determined using the first law of thermodynamics (Eq (1)).W is the mechanical work generated by the moving walls, Q is the total heat, m the mass and H is the enthalpy. Subscript i indicates an intake flow, c indicates a charging pipe flow and e indicates an exhaust flow. Then:i c e dm dm dm dm =++(3)Each mass flow rate is determined using a valve boundary condition (See corresponding section of this paper). A classical numerical integration technique then yields the new total mass inside the cylinder.The total heat Q is the sum of a combustion heat c Q and convective heat transfer through cylinders walls w Q .c w Q Q Q δδδ=+(4)w Q δ and c Q δ are respectively determined using the Woshni (13) and Wiebe (14) models. These classical correlations are notdetailed in this paper. Re-arranging Eq (1) finally gives:()p k k v vpdV Q c T dm c TdmdT mc δ-++-=∑(5)A numerical integration gives the new temperature of the cylinder. The volume is determined from the engine geometry and crankshaft position (15). The pressure is then calculated using ideal gas law.Page 4 of 16 ONE-DIMENSIONAL COMPONENTS OF THE ENGINE MODEL FUNDAMENTALSFigure 2: Control VolumeFlows, in each pipe, are assumed to be mono dimensional. The ideal compressive gas is assumed to be inviscid. Three conservation laws are written for a control volume, respectively the mass, momentum and energy. Figure 2 shows the flow of an ideal compressible gas through an infinitesimal section of pipe. ,,,,p u t x ρand F are respectively the pressure, the density, the velocity , the time, the space and the cross section area .Continuity equation: The rate of change of mass within the control volume is equal to net mass flow rate through the element. This principle leads to (16) (17):0u u dF t x F dxρρρ∂∂++=∂∂(6) Momentum equation: The rate of change of momentum within the control volume is equal to the sum of the forces applied on thecontrol volume. In order to model the friction between the flow and the wall, a shear stress w τ, opposed to the flow, is introduced. Expressing the shear stress in terms of the pipe wall frictionf gives 212w u f τρ=. D is the diameter of the pipe. 220u u p u dFG t x F dxρρρρ∂∂++++=∂∂(7) 14||2G u u f D=(8)Energy equation: The energy equation is derived from the first law of thermodynamics applied to the control volume. Heat transferper unit mass of gas q has been introduced to take into account the heat transfer between the pipe and the fluid. q is determined from an approximate treatment (16), where w T and g T are the temperature of the pipe wall and gas respectively. 0e and 0h arerespectively the stagnation specific energy and enthalpy.0000e uh uh dFq t x F dxρρρρ∂∂++-=∂∂(9) 2()1w g fu Rq T T D γγ=--(10)Page 5 of 16 TWO-STEP LAX-WENDROFF SCHEME + T.V.DFigure 3: 2 steps Lax Wendroff (Richtmeyer) stencilThe three previous governing equations can be favorably rewritten in vectorial form:()()0W F W C W t x∂∂++=∂∂(11) 0[,,]T W u e ρρρ= is the state vector of conservative variables: mass, momentum and total energy20()[,,]T F W u u p uh ρρρ=+ is the flux vector. Vector ()C W is the correction vector. It takes into account section variation,pipe wall friction and wall heat transfer:200(ln )()u d F C W u p G dx uh q ρρρρρ⎡⎤⎡⎤⎢⎥⎢⎥=++⎢⎥⎢⎥⎢⎥⎢⎥-⎣⎦⎣⎦(12) Eq (11) can be solved by a two-step Lax-Wendroff method (18), as shown in Figure 3. First step :12111121()())224 n n n n n n ni i i i i i i t t WW W F W F W C C x +++++∆∆⎡⎤⎡⎤⎡⎤=+---+⎣⎦⎣⎦⎣⎦∆(13) 12111121()()) 224n n n n n n ni i i i i i i t t WW W F W F W C C x +----∆∆⎡⎤⎡⎤⎡⎤=+---+⎣⎦⎣⎦⎣⎦∆(14)Second step :111221122() ())n n n nn ii i i i t W W F W F W tC x ++++-⎡⎤∆=---∆⎢⎥∆⎣⎦(15)Page 6 of 16 Because the LW2 scheme is not Total Variation Diminishing (T.V.D) (19) spurious oscillations appear at shocks anddiscontinuities. A flux limiter has to be added to the original LW2 scheme. The simplified T.V.D scheme of Davis (20) has been used. The advantages of this scheme are that it is symmetrical (i.e. does not depend on the direction of travel of the characteristics), and that it is easily implemented in the calculation process:111221111111111222222()())()()()()()()n n n nn n n n nii i i i i i i i i i i i i i i i t W W F W F W tC K r K r W W K r K r W W x +++++--++--++--+-++--⎡⎤⎡⎤⎡⎤∆=---∆++-++-⎢⎥⎢⎥⎢⎥∆⎣⎦⎣⎦⎣⎦(16)The functions i K +-and ir +-are not detailed is paper, and the reader is referred to the original paper (20).OPEN END BOUNDARY CONDITIONFigure 4: Open End Boundary ConditionThe open end boundary condition used at the pipe ends is Method Of Characteristic based and uses the C.I.R Method (21). Figure 4 depicts the general context of this boundary. Non dimensional notations from Benson are used (16; 17). Subscripts ‘ref ’ and ‘ext ’ are respectively the reference and the exterior state.Outflow from pipe to cylinder:In this case, the characteristic line C +and path-line 0C exist as depicted in Figure 4.Three primitive quantities at the boundary are unknown :1n iρ+,1n iu + and1n i p +.First, using the CIR method, the non-dimensional Riemann invariant along the C +characteristic line is determined111122n nn n in L L i i refA U A U a αγγλ++--==+=+(17)If the flow is subsonic, the pressure at the boundary is assumed to be equal to the exterior pressure1n i ext p p +=(18)If the flow is subsonic, then contraction downstream the throat is assumed to be isentropic:2111n n i iext ext pa p a γγ++-⎡⎤=⎢⎥⎣⎦(19)Re-arrangement of Eq.(18) and Eq.(19) gives :Page 7 of 16 1n i ext A A +=(20)Using equations (17)(18)(20) the subsonic state at the boundary condition can be solved. The obtained solution has to be checked as a subsonic one. In the contrary case, the constant pressure and homentropic contraction hypotheses are no longer valid. The flow is now assumed to be sonic at the throat:11n n i i U A ++=(21)The homentropic flow along the 0C path line gives :()()11n ni s n n isp p γγρρ++=(22)Using equations (17)(21)(22) sonic state at the boundary condition can be solved.Inflow from cylinder to pipe:In this case, the pathline 0C no longer exists. Three primitive quantities at the boundary are unknown: 1n i ρ+,1n iu + and1n i p +.If the flow is subsonic, the characteristic line C +exists, then :111122n nn n in L L i i refA U A U a αγγλ++-==+=+(23)Gas contraction upstream the throat is assumed to be homentropic:221111()n n i ii ext ext pa A p a γγγ++--⎡⎤==⎢⎥⎣⎦(24)The steady state total energy conservation equation gives :12121()()2n n ext i i A A U γ++-=+(25)Using equations (23)(24)(25) the inflow subsonic state at the boundary condition can be solved. The solution obtained has to be checked as a subsonic one. In the contrary case, the characteristic line C + is no longer valid, and Eq (23) cannot be used and must be replaced by the sonic flow condition at throat, given by Eq (21). The sonic inflow state is then computed using equations (21)(24)(25).Page 8 of 16 Entropy correction for gas inflowing into pipe:Figure 5: (a) Benson Entropy Level (b) Entropy correction at BoundaryIn the case of gases inflowing into the pipe at the boundary condition, the M.O.C. resolution used in the previous section assumes an homentropic flow. This assumption means, firstly, that gases located in the first node of pipe are at the same entropy level as those in the cylinder, and secondly, that there is no entropy creation across the valve throat. Needless to say, these ideal conditions are never encountered in a real flow, thus making entropy correction necessary. The change of Riemann variable along the characteristic line C - due to a change of entropy level is defined by the following equation, see Benson (16):,,,,2in c out c Ac An Ain c in n A Ac A A dA d AA A λλλλλ+⎛⎫⎛⎫-=-=⎪ ⎪⎝⎭⎝⎭(26)As the three quantities ,in c λ, ,out c λ and Ac A refer to a new time step level 1n +, Eq (26) has to be solved by an iterativemethod. In chapter 7 of his book, Benson (16) proposes an algorithm to converge previous equation, based on the estimated entropy level Ac A .INTERMEDIATE VOLUME VALVE BOUNDARY CONDITIONFigure 6: Intermediate Volume Valve Boundary ConditionThe original MOC valve boundary condition (see Figure 6) has been defined and experimentally validated in a previous work from authors (12) . The main interests of this boundary condition are first, to avoid any convergence problem or non-physical shock that may be encountered while using of the classical constant pressure model from Benson, and second, to obviate the use of a Coefficient of discharge. See (12). The boundary is constituted by an intermediate volume that connects the pipe and the cylinder. This volume exchanges mass through two ports. The first one is a MOC Open End Boundary Condition (see previous section) thatconnects the intermediate volume and the pipe. The second one is the valve port that connects the intermediate volume to the cylinder.Page 9 of 16 Mass flow rate through the valve port:The mass flow rate through the valve port is calculated with the classical Barre de Saint Venant equation, governed by the pressure ratio at the throat. Four cases must be distinguished. The flow can be in the forward or the reverse direction (back flow) and it can be sub or supersonic. Eq (29) presents the critical pressure ratio (c r ) at the throat which determines the type of flow. If thepressure ratio at the throat, defined as dpup r p =, is lower than the critical ratio, the flow is supersonic, otherwise the flow is subsonic. Because the pressure ratio p r must be lower than unity, u p and d p are defined as:1( ,)n u iv ext p max p p +=(27) 1( ,)n d iv ext p min p p +=(28)121c r γγγ-⎛⎫= ⎪+⎝⎭(29)Eq (30) and Eq(31)give the mass flow rate at the throat respectively for a subsonic and a sonic flow. v Cd is a possible dischargecoefficient at the valve if necessary:1vd v t u dm p Cd F dtp γ⎛⎫=⎪⎭12(1)0.521v v t dm Cd F dt γγγ+-⎛⎫= ⎪+⎝⎭(31)The sign ofvdm dtmust be adapted to the direction of the flow: the sign is set positive if the flow enters the intermediate volume. Mass flow rate through pipe port:The mass flow rate through the pipe port of the intermediate volume (p subscript) is determined using the Open End Boundary Condition presented before. This mass flow rate is determined using Eq (32). It can be seen that no discharge coefficient is used at this port:11**p n n i i i dm F u dtρ++=(32)Iterative resolution scheme:Because each mass flow ratep dm dtandv dm dtdepends on the pressure level of the intermediate volume at the new step time (1n iv p +), an iterative resolution is needed. Reader is pleased to refer to original paper for details (12).Page 10 of 16EXPERIMENTAL VALIDATIONTable 1 – Prototype engine dimensionsThe pneumatic hybrid engine used for experiments is a prototype single cylinder, 347 cm 3 displacement, 4-Stroke, Spark-Ignition, with hemispherical cylinder head and flat piston. Table 1 gives the general engine dimensions and Table 2 gives the specifications of the valves, pipe and actuators. In-cylinder pressure is measured by a piezoelectric pressure sensor. The intake, charging and exhaust port pressures are measured by three absolute piezo-resistive sensors. Intake air port temperature is measured by a Pt100 sensor, and exhaust and charging port temperatures are measured by two K-thermocouples. The intake and charging mass flow rates are measured with two Mass flow controllers. The exhaust pressure is regulated by a control valve. The intake and charging air can be warmed up by two resistive heaters. Two plenums of respectively 6 and 30 liters volume are mounted on intake and exhaust lines. Temperature controls are implemented on both water and oil circuits. Two operating modes of the engine are studied: 4-stroke Pneumatic Pump and 4- stroke Pneumatic Motor.Table2 –Valves and pipes specificationsEach simulation performed included 4 complete engine cycles, starting from null speed steady state inside each pipe. All the numerical results presented were taken from the third simulated cycle, to ensure that non-stationary states were sufficiently present in each pipe. The discharge coefficient of all the valves was set to unity. Woshni's heat loss coefficient 1C (13) was fitted on pure compression measurements. It should be noted that a strong hypothesis has been made by supposing a constant wall temperature along a given pipe (intake, exhaust and charging). Each pipe is modeled as a straight duct without any sudden enlargement and section change.Table 3 –Operating parametersFour-Stroke Pneumatic Pump mode :Table 4 –Numerical results for 4-stroke pneumatic pump modeby the entire engine model. Figures 7 and 8 respectively display the p-V diagram and the charging port pressure versus Crack Angle.Figure 7: pV diagram- Pneumatic 4-stroke pumpFigure 8: Charging port pressure versus Crank Angle- Pneumatic 4-stroke pumpThe simulated p-V diagram has a general shape close to the measured one, a point that is confirmed by the very low relative error ( -1.27 %) between the measured and simulated values of the indicated WorkiW . The simulated value of charging air mass per cycle exhibits a +4.87 % relative error compared to the measured one. This result can be considered excellent, given that the discharge coefficient was set to 1, and never have been fitted.Page 11 of 16Page 12 of 16 Analysis of the charging port pressure vs. crank Angle (see Figure 8) shows that the simulated pressure wave is very close to the measured one in terms of amplitude and timing. This is a key point with regard to our objective, which is to be able to predict the optimum timings of opening and closing of the charging valve.Figure 9 : Intake and exhaust charging port pressure versus Crank Angle- Pneumatic 4-stroke pumpThe simulated intake air mass presents a deviation and a relative error respectively of +61 mg/cycle and 19.3 %, in respect to measurement. This poor result is due an inappropriate size and form of the intake plenum. Thus the waves existing inside the upstream and downstream pipes of the plenum were not sufficiently decoupled. This affirmation is clearly highlighted by Figure 9. The simplest way to solve this problem would have been to improve the plenum, in order to sufficiently decouple the upstream and downstream waves.Before regarding to the exhaust air mass per cycle, it is important to note that to obtain a correct simulated value, it is necessary to have accurate values for intake and charging air mass per cycle. All errors are echoed on exhaust air mass value, because 0intake charging exhaust m m m ++=. When this fact is taken into account, the observed deviation of -50 mg/cycle between simulated and measured exhaust air mass is logical, because of the deviations observed on intake and charging air mass respectively of +61 mg/cycle and -11 mg/cycle. Moreover, an analysis of the exhaust port pressure versus Crank Angle (Figure 9) shows that the amplitude and decay of the pressure wave is correctly described by the model, but that a phase shift is gradually installing. Authors assume that this phenomenon is due to a non-negligible convective heat transfer existing along the charging pipe which has not been taken into account by the model.Because the main goal was to correctly modelize the charging pipe behavior, authors have not spent more time to solve those issues.Four-Stroke Pneumatic Motor mode:Table 5 –Numerical results for 4-stroke pneumatic motor modePage 13 of 16Figure 10: p-V diagram- Pneumatic 4-stroke motorFigure 11: Charging port pressure versus Crank Angle- Pneumatic 4-stroke motorTable 5 presents the numerical results for a representative measured cycle on the test bench, and numerical results produced by the entire engine model. Figures 10 to 12 respectively display the p-V diagram, the charging, the intake and the exhaust port pressure versus Crack Angle.Figure 12: Intake and exhaust port pressure versus Crank Angle- Pneumatic 4 stroke motorPage 14 of 16 First, an analysis of the charging port pressure vs. Crank Angle (Figure 11) shows that the simulated pressure wave is very close to the measured one in terms of amplitude and timing. This is a key point for a future valve actuation timings optimization. Moreover, the analysis of the charging air mass per cycle shows a relative error of only -3.89% between air simulated and measured is observed (Table 5). Analysis of Figure 12 leads to similar conclusions as for the pneumatic pump mode. The intake plenum is inappropriate and convective heat transfer along the exhaust pipe cannot be neglected.Surprisingly, a comparison of the shapes of the measured and simulated p-V diagrams leads to less satisfactory results than for the pneumatic pump mode. The observed relative error of -17.98% between measured and simulated values of the indicated Work i W confirms this point. Visually, it is obvious that the variation occurs during the opening of the charging valve. The measured in-cylinder pressure is higher than the simulated one. Thus more energy than expected has been transferred to the in-cylinder gas. Authors conjecture that the opening of the charging valve, given of the high pressure ratio that exists between the pneumatic charging port and the cylinder, significantly changes the in-cylinder internal aerodynamic structure that produces a positive convective heat transfer process.SUMMARY/CONCLUSIONSThe present work proposes a complete 1D engine model for pneumatic-combustion hybrid engine. A comparison of experimental and simulated results with no discharge coefficient has shown good results, at the charging port, in terms of mass flow rates per cycle, pressure wave timings and amplitude for both investigated pneumatic modes. For the pneumatic 4-stoke pump mode, the predictions of Indicated Work and p-V diagram were satisfactory. But, in the special case of the pneumatic 4-stroke motor, the indicated work was under estimated by the model. The model is clearly underestimating the energy transfer during the pneumatic charging process. It has been supposed that this phenomenon is due to a convective heat transfer process created by a strong in-cylinder air motion during the pneumatic charging. Future works has to experimentally investigate the in-cylinder aerodynamic structure and the heat transfer process during the charging valve opening.As a final conclusion, the gas dynamic modeling into the charging pipe and mass flow rates through valves has been experimentally validated that allows a future charging valve timing optimization work.REFERENCES1. New cycles for automobile engines. SCHECHTER, M. 1999, SAE Technical Paper, Vols. 1999-01-0623.2. Thermodynamic cycles for a new hybrid pneumatic-combustion engine concept. HIGELIN, P. and CHARLET, A. 2001, SAE Paper, Vols. 2001-09-23.3. Modelling and optimizing two- and four-stroke hybrid pneumatic engines. DONITZ, C., et al. 2009, IMechE Part D - Journal of Automobile Engineering, Vols. Vol. 223, No. 2, pp. 255-280.4. Realizing a concept for high efficiency and excellent driveability: The downsized and supercharged hybrid pneumatic engine. DONITZ, C., et al. 2009, SAE paper, Vols. 2009-01-1326.5. Energy Management Strategies for a Pneumatic-Hybrid Engine Based on Sliding Window Pattern Recognition. IVANCO, A., et al. 2009, Oil and Gas Science and Technology, Vol. DOI: 10.2516/ogst/2009045.6. Vehicle Driving Cycle Simulation of a Pneumatic Hybrid Bus Based on Experimental Engine Measurements. TRAJKOVIC, S., TUNESTAL, P. and JOHANSON, B. 2010-01-0825 , 2010, SAE Technical paper, Vols. 2010-01-0825.7. Well-To-Wheels Use and Greenhouse Gas Emissions of Plug-in-Hybrid Electric Vehicles. ELGOWAINY, A., et al. 2009, SAE paper, Vols. 2009-01-1309.8. Investigation of Different Valve Geometries and Valve Timing Strategies and their Effect on Regenerative Efficiency for aPneumatic Hybrid with Variable Valve Actuation. TRAJKOVIC, S., TUNESTAL, P. and JOHANSSON, B. 2008, SAE Paper, Vols. 2008-01-1715.。