Optimization of electrochemical treatment
堆肥腐殖酸形成及其电化学活性
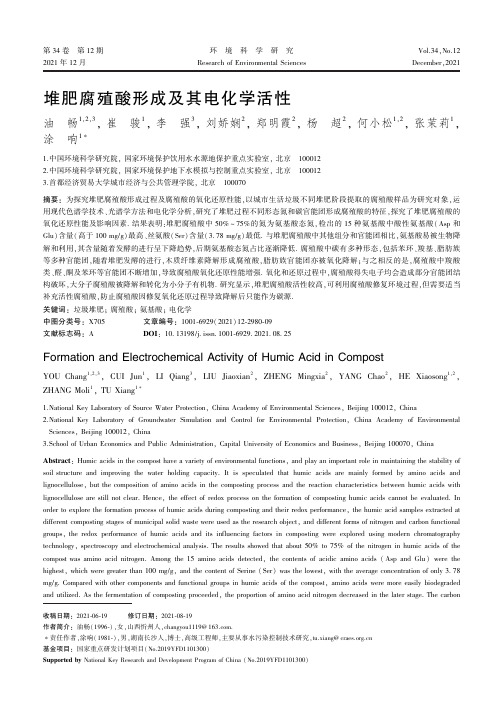
补充活性腐殖酸ꎬ防止腐殖酸因修复氧化还原过程导致降解后只能作为碳源.
关键词: 垃圾堆肥ꎻ 腐殖酸ꎻ 氨基酸ꎻ 电化学
中图分类号: X705 文章编号: 1001 ̄6929(2021)12 ̄2980 ̄09
prevent humic acids from being used just as a carbon source after the degradation of the redox process. The findings above provide a
theoretical basis for improving the fertilizer efficiency and environmental remediation performance of composting products.
lignocelluloseꎬ but the composition of amino acids in the composting process and the reaction characteristics between humic acids with
lignocellulose are still not clear. Henceꎬ the effect of redox process on the formation of composting humic acids cannot be evaluated. In
增加以及垃圾分类的有效推进ꎬ城市生活垃圾中易腐
剧
[1]
. 堆肥是一 种 生 物 转 化 技 术ꎬ 可 以 实 现 易 腐 垃
Investigation, Analysis and Optimization of Exothermic Nitrations in Microreactor Processes
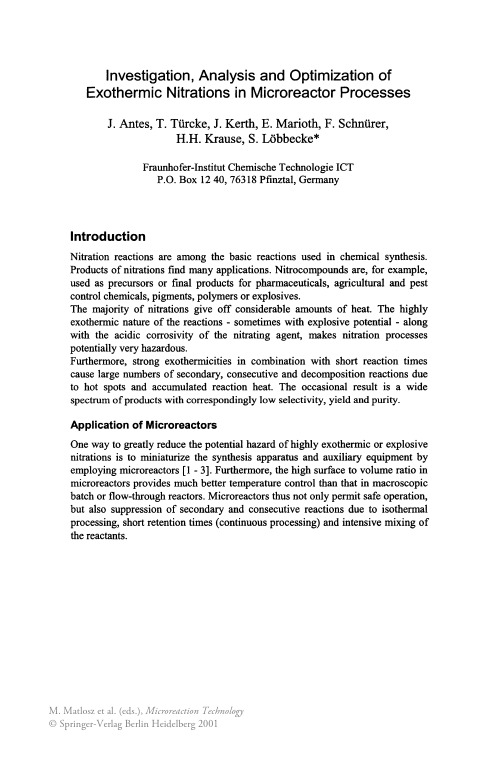
Two different types of microreactors were used for the nitration experiments. A commercially available silicon micromixer based on a microfluidic split-andrecombine structure (MiMoCo GmbH, IlmenauiGermany) and a microreactor array made of glass containing 20 reaction channels in parallel with integrated educt mixing zones and cooling structure (development in cooperation with mgt mikroglas technik AG, Mainz/Germany) [2, 3]. Since nitrating agents are highly corrosive media only resistant materials can be applied for such reactions. Silicon, glass and titanium were proved to be suitable for nitration processes. Microstructured devices made of stainless steels did not withstand such corrosion processes. Different nitrating agents were used for the microreaction experiments. Besides concentrated and fuming nitric acid (65% resp. conc. RN0 3), nitrating acid ("mixed acid": RN0 31H2S04 = 1: 1 - 6: 1) and dinitrogen pentoxide (N205) as a less acid nitrating agent were used. N20 5 was either dissolved in CH2Cl 2 or used as a gaseous reactant (in-situ production: N20 4 + 0 3). Nitrating agents were used in up to 6-fold excess. Ureas (dissolved in dichloromethane) and nitrating agents (liquid or dissolved) were supplied by pulsation-free pumps, mixed inside the microreactors (resp. micromixers) and then passed through reaction capillaries (e.g. PTFE tubes) of different lengths representing different retention times (0.6 s - 82 s). Nitrations were carried out in a continuous mode at defined temperatures between O°C and 20°C. The reaction mixture eluting out of the microreaction system was quenched in ice water and/or cold dichloromethane, extracted and passed to NMR, MS, IR, HPLC or GC-MS for analysis. For a more effective investigation and optimization of nitrations analytical devices and sensors for process control (like temperature monitoring, flow control, etc.) were adapted to the microreaction processes. FTIR microscopy was applied for the online monitoring of nitrations in silicon microreactors. Due to a high spatial resolution of this analytical method (~ 10 flm) educts and products of nitrations can be identified and distinguished at different positions inside the microreactor [5]. Both intermediates and final products of nitrations can be IR spectroscopically detected by focusing the IR
特定谐波消除及优化脉宽调制单相整流器的研究
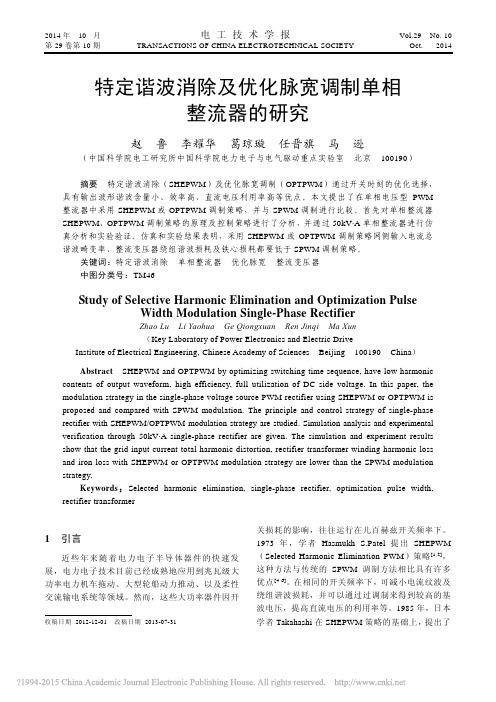
z
2 n x 2 s 2 n
( 1)
y
图1 Fig.1 单相电压型 PWM 整流器主电路拓扑 The topology of single-phase voltage source PWM rectifier
2014 年 10 月 第 29 卷第 10 期
电 工 技 术 学 报
TRANSACTIONS OF CHINA ELECTROTECHNICAL SOCIETY
Vol.29 No. 10 Oct. 2014
特定谐波消除及优化脉宽调制单相 整流器的研究
赵 鲁 李耀华 葛琼璇 任晋旗 马 逊
北京 100190) (中国科学院电工研究所中国科学院电力电子与电气驱动重点实验室 摘要
式中, n 为谐波次数; 1 为基波频率。 定义电流谐波畸变率为
2 THDi I 0
1, 2,…, 7 是七个可以独立控制的开关角
变量,对图 4 中输出电压 U ab 进行傅里叶分解得
U ab
=
n 2,3,
2 In
( 8)
如果不考虑电流的直流分量,则
1 THDi 1 L un n n 2,3,
s
2 s n 2
x
( 2)
arccos
x 2 x y2
( 3)
2.2
单相电压型 PWM 整流器控制策略 ′ 单相 PWM 整流器控制系统框图如图 2 所示 ( us
式中, y 可以无差地跟踪 x 中角频率为 n 的正弦信 , z 与 y 的幅值、频率相 号, z 滞后 y 的角度为 90° 同。通过 y 和 z 的瞬时值可以得到 x 中角频率为 n 正弦信号的瞬时角度。
脉冲频率对电沉积CoFe合金镀层组织和磁性能的影响
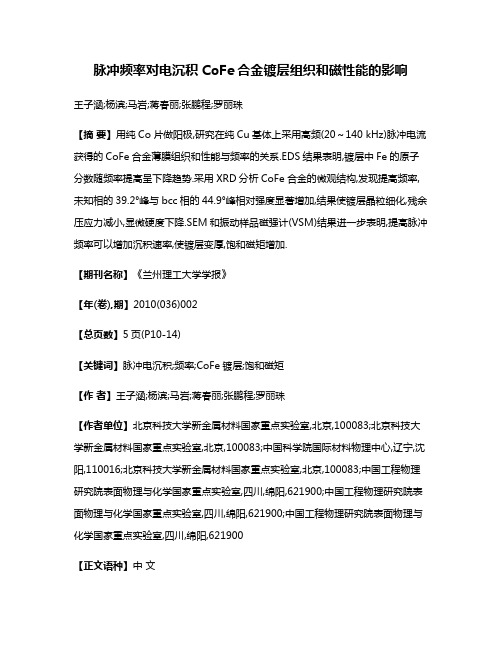
脉冲频率对电沉积CoFe合金镀层组织和磁性能的影响王子涵;杨滨;马岩;蒋春丽;张鹏程;罗丽珠【摘要】用纯Co片做阳极,研究在纯Cu基体上采用高频(20~140 kHz)脉冲电流获得的CoFe合金薄膜组织和性能与频率的关系.EDS结果表明,镀层中Fe的原子分数随频率提高呈下降趋势.采用XRD分析CoFe合金的微观结构,发现提高频率,未知相的39.2°峰与bcc相的44.9°峰相对强度显著增加,结果使镀层晶粒细化,残余压应力减小,显微硬度下降.SEM和振动样品磁强计(VSM)结果进一步表明,提高脉冲频率可以增加沉积速率,使镀层变厚,饱和磁矩增加.【期刊名称】《兰州理工大学学报》【年(卷),期】2010(036)002【总页数】5页(P10-14)【关键词】脉冲电沉积;频率;CoFe镀层;饱和磁矩【作者】王子涵;杨滨;马岩;蒋春丽;张鹏程;罗丽珠【作者单位】北京科技大学新金属材料国家重点实验室,北京,100083;北京科技大学新金属材料国家重点实验室,北京,100083;中国科学院国际材料物理中心,辽宁,沈阳,110016;北京科技大学新金属材料国家重点实验室,北京,100083;中国工程物理研究院表面物理与化学国家重点实验室,四川,绵阳,621900;中国工程物理研究院表面物理与化学国家重点实验室,四川,绵阳,621900;中国工程物理研究院表面物理与化学国家重点实验室,四川,绵阳,621900【正文语种】中文【中图分类】TG132.2硬盘驱动器的写头材料中,CoFe合金具有比Fe-Ni合金更优异的磁性能,随着存储密度的增加,Ni80Fe20合金已经无法满足在高矫顽力磁介质上高频写入数据的要求,因此开发具有更高饱和磁感应强度的CoFe合金成为当务之急[1-3].电沉积是一种操作简单,成本低廉的薄膜材料制备方法.脉冲电沉积依据的电化学原理是:利用电流(或电压)脉冲的张弛增加阴极的活化极化和降低阴极的浓差极化,以达到改善镀层物理化学性能的目的[4].镀液成分、温度、pH值及电流密度对脉冲电镀CoFe合金的沉积速度、镀层组织和力学性能的影响已有大量报道[5-6],而高频脉冲下频率对CoFe合金镀层化学成分,组织和磁性能的影响鲜有报道.作者[7]在高频脉冲镀Ni的研究中发现,提高脉冲频率,能细化镀层晶粒,提高致密度,增强密排面择优取向和显著改善力学性能,因此本文研究脉冲频率(20~140 kHz)对电沉积CoFe合金镀层组织及磁性能的影响,探索制备高性能CoFe合金薄膜的最佳工艺.1 实验材料及实验方法阳极用纯Co片,厚度为0.3 cm,长度和宽度分别2 cm和1 cm.阴极为纯铜片,厚度0.1 cm,长度和宽度分别2 cm和1 cm.500 mL玻璃烧杯用做镀槽,阴阳极板在镀槽中保持平行,极间距约为6 cm.每次实验需配制100 mL新镀液,溶剂用蒸馏水,化学试剂用分析纯,具体成分如下:CoSO4◦7H2O(0.05 mol/L),FeSO4◦7H2O(0.09 mol/L),H3BO3(0.4mol/L),(NH4)3C6H5O7(1 g/L),C12H25SO4Na,少量.电镀过程工艺及参数为:镀液pH值4.0;恒温水浴加热,温度35 ℃;磁力搅拌,转速300 r/min;采用恒电流方式沉积,平均电流密度10 mA/cm2;占空比20%;沉积时间1 h.采用PHILIPS APD-10 X射线衍射仪(XRD)分析CoFe合金镀层微观结构,D/max-2500型高功率多晶X-射线衍射仪(XRD)分析薄膜的残余应力,LDJ 9600强磁场振动样品磁强计(VSM)测量合金表面平行方向磁性能.采用ZEISS SUPRA55型场发射扫描电镜(FE-SEM)观察镀层显微组织和表面形貌,并用电镜配置的X射线能谱仪(EDS)在镀层表面取上中下3点进行成分分析.采用JEOL JSM-6390扫描电子显微镜(SEM)观察镀层截面组织形貌,画3条垂直于镀层的平行线,用电镜标尺测量镀层厚度,结果取平均值.采用HVS-1000数字显微硬度仪测量镀层显微硬度,在镀层表面随机选取4点,结果取平均值.2 实验结果及分析2.1 脉冲频率对CoFe镀层组织及晶粒择优取向的影响脉冲频率对CoFe合金成分的影响如表1所示,20 kHz频率沉积时,镀层中Fe原子分数是60.5%,而升至140 kHz时则下降到49.7%.研究发现,在保持电流密度恒定的情况下,阳极Co片的溶解量不随频率发生变化,并且新生成的Co2+摩尔数也远低于原溶液中Co2+的摩尔数,因此认为镀液中钴和铁的摩尔比基本保持不变,但由于这部分离子在搅拌作用下,随镀液从阳极迁移至内扩散层外边界,导致Co2+数量较Fe2+局部占优;因而镀层中Co原子分数比较Fe高.而提高脉冲频率又会增加阴极过电势,结果向阴极迁移的Co2+数量随之增多,使镀层中Co和Fe的原子比进一步提高.表1 不同脉冲频率下沉积的CoFe合金成分Tab.1 Composition of CoFe alloy films deposited with various pulse frequency?一般认为,具有良好软磁性能的CoFe和CoFe基合金材料都由bcc和fcc双固溶体相组成,具体相组成由各相所含元素的原子比和沉积条件决定[8].图1为不同脉冲频率下CoFe合金镀层XRD衍射图谱.可见,在20 kHz沉积的CoFe合金镀层,未发现fcc(111)峰,其最强衍射峰位于44.9°处 ,是bcc α-Fe 相,同时观察到微弱的未知相39.2°峰,说明其为多相合金.而在140 kHz电镀后的合金镀层中,虽仍未观察到fcc(111)衍射峰,但形成鲜对比的未知相的39.2°峰成为最强峰,而bcc(110)峰则成为弱峰.图1中主要强峰相对衍射强度随频率增加发生规律性变化的主要原因是:1)镀层中Co和Fe的原子比发生变化.一般情况下,随着镀层中Fe的原子分数逐渐减少,合金中bcc相的数量会增加,而fcc相会减少[9];2)阴极极化逐渐增大.通常在极化较小条件下共沉积,形成的是单相固溶体或过饱和固溶体,而在较高极化时,则形成复相合金[4].由于高频脉冲会产生较大的阴极极化,因此沉积层易生成复相合金和非平衡相,初步分析认为39.2°峰是在上述2种因素共同作用下生成的过渡相的一个衍射峰.与其他研究者观察到的不一致.Zhang[9]等人的研究中采用低频脉冲沉积CoFe合金镀层,在Fe原子分数29%~69%时,主要由bcc的α-Fe和fcc的γ-Fe/α-Co固溶体相组成,当Fe原子分数超过69%时全部为fcc相.而Armyano[10]在沉积CoFe合金膜时发现,Fe原子分数在32%~75%时,是单相的 bcc结构.因此,通过改变频率,可以在相似镀液中采用高频脉冲沉积出具有特定相结构的复相合金. 图1 不同脉冲频率下CoFe合金镀层XRD衍射图Fig.1 XRD patterns of CoFe alloy films formed with various pulse frequency2.2 脉冲频率对CoFe镀层组织形貌的影响对不同频率沉积的CoFe合金镀层进行表面FESEM 观察,如图2所示.可以发现,频率增加后,CoFe合金镀层中晶粒有细化的趋势,主要原因有2点.Allen Bailey[11]在研究CoFe合金沉积时认为阳极溶解对镀层形貌具有非常重要的影响.高频脉冲沉积过程中,提高频率,镀液中会有较多的Co2+在镀层表面放电生成原子,结果未知相与bcc相相对数量因此发生变化,进一步影响到电结晶过程,促使各相长大竞争加剧,因而细化了整个镀层晶粒.另外,由于高频脉冲有利于提高阴极过电势,通常认为这会细化晶粒,因此,提高脉冲频率有利于生成细小均匀的组织.2.3 脉冲频率对CoFe合金沉积速率的影响CoFe合金镀层截面SEM组织形貌如图3所示,可见镀层连续致密,厚度较为均匀.图3a为20 kHz沉积的Co40Fe60合金,镀层平均厚度约为2.10 μ m.图3b为80 kHz时沉积的Co43Fe57合金,厚度约为5.27 μ m.图3c为140 kHz时沉积的Co50Fe50合金,厚度约为9.94 μ m.易见,随着脉冲频率提高,沉积1 h后的镀层厚度显著增加.由电化学[12]基本定义可知,脉冲平均电流密度jm与频率f有如下关系式:式中:jm为脉冲平均电流密度;jp为脉冲峰值电流密度;ton为导通时间;toff为关断时间;脉冲周期θ=ton+toff;脉冲频率f=1/θ;ν为脉冲导通时间与脉冲周期之比,即占空比.由式(1)可知,保持jm和ν不变,则jp也将保持恒定.因此脉冲频率 f增加后,ton必然减小.脉冲沉积过程中,电极表面存在双扩散层,在靠近阴极的内扩散层中,金属离子浓度随频率而波动.由Fick第二定律可推导出电沉积过程扩散层厚度:式中:δp为内扩散层厚度;D为镀液的扩散常数.式(2)表明,随着ton减小,扩散层厚度δp也随之减小.由电化学理论可知,随着扩散层的减薄,放电离子向电极表面的扩散阻力相应减小,因而扩散速度加快,阴极电流效率提高,镀层沉积速率增大.由法拉第定律可推出镀层厚度计算公式:图2 不同脉冲频率下CoFe合金镀层表面组织形貌Fig.2 FE-SEM image of CoFe alloy films formed with various pulse frequency图3 不同脉冲频率下CoFe合金镀层截面组织形貌Fig.3 Cross-sectional morphology of CoFe alloy films formed with various pulse frequency式中:δ为镀层厚度,mm;C为电化当量,g/(A◦h);d为沉积金属的比重,g◦cm-3;Im为电流密度,A/dm2;η为电流效率,%.由式(3)可知,电沉积过程中,保持其他参数恒定,提高电流效率η,镀层厚度将显著增加.因此,提高脉冲频率可提高CoFe合金的沉积速率.2.4 脉冲频率对CoFe合金镀层残余应力的影响用X射线应力分析法测量CoFe合金薄膜的平均残余应力,尽管合金中Co和Fe原子比发生变化,但通常情况下,纯Fe和Co的弹性模量较为接近,因此合金薄膜的弹性模量E取平均值209 GPa,泊松比μ可取0.29,用经典的sin2ψ法分析应力,采用重心法定峰.结果如下:20 kHz薄膜的残余应力为-590.2 MPa,80 kHz的为 -406.9 MPa,140 kHz的为-366.9 MPa.结果表明,脉冲频率增加后,镀层的宏观压应力减小.ZHANG T Y[13]等用压痕法测量沉积在Si表面的SiO2和Cr膜时发现,其应力不随膜的厚度发生变化.因此,应力随频率提高而减小的原因,分析认为,主要是高频脉冲沉积的镀层晶粒更细小、组织更均匀.2.5 脉冲频率对CoFe镀层磁性能的影响CoFe合金的磁性能主要取决于合金成分及结构,另外,镀层厚度对磁性能也会有一定影响.图4为不同脉冲频率沉积的CoFe合金镀层的磁滞回线,可见,20 kHz沉积的合金饱和磁矩为41.77×10-6A◦m2,80 kHz的为228.6×10-6A◦m2,140 kHz的为316.1×10-6A ◦m2,由此可知,饱和磁矩随脉冲频率增加而增大.主要是提高频率,增加镀层的沉积速率,使膜厚显著增加.饱和磁感应强度只与成分、原子结构、组成合金各相的数量比有关.因此沉积频率提高对CoFe合金镀层成分的改变影响镀层的饱和磁感应强度.经过计算,Co40Fe60合金的饱和磁感应强度可以达到1.98 T,Co43Fe57的为2.21 T,Co50Fe50为2.32 T.比Zhang[9]等制备的Co65Fe35合金的1.96 T略高,这表明用高频脉冲沉积的CoFe合金薄膜具有较高饱和磁感应强度.众所周知,矫顽力是结构敏感参数,与合金成分,晶粒形状及尺寸以及内应力等有关.如前所述,在固定其他沉积参数的情况下,改变脉冲频率使镀层组织更均匀,内应力更小.通常认为,随着内应力减小,磁性材料的矫顽力会减小,Kozlov[14]根据实验结果推导了纯铁的应力和矫顽力关系式为CoFe合金由于磁弹性系数以及成分变化,矫顽力与应力没有呈现纯铁的那种线性关系[14].虽然高频脉冲有助于细化晶粒,使组织更均匀,但在晶粒小于一定尺度后,反而由于晶界总长度增加阻碍了磁畴的迁移或旋转,增加了镀层的矫顽力[15].因此在双重因素作用下,CoFe合金膜的矫顽力从20 kHz时的7957.5 A/m减小到80 kHz 时的1512.0 A/m,随后又升高到140 kHz的2626.1 A/m,呈非线性变化,总趋势是140 kHz脉冲比20 kHz脉冲沉积的镀层矫顽力小.为了阻止阳极附近的Fe2+氧化为Fe3+,从而恶化合金的磁性能,镀液中加入柠檬酸铵,少量的添加剂是不会提高矫顽力的.其中80 kHz时沉积的 Co43Fe57合金膜的矫顽力较Zhang[9]等沉积的合金(636.6 A/m)和Oscar[16]沉积的合金(1193.7 A/m)高一些,但基本可以满足要求,至于矫顽力较大的Co40Fe60和Co50Fe50合金欠妥需要进一步退火处理.2.6 脉冲频率对CoFe镀层硬度的影响图4 不同脉冲频率下沉积的CoFe合金膜磁滞回线Fig.4 In-plane hysteresis loop of CoFe films deposited at various pulse frequency镀层的显微硬度主要由沉积层组织和结构决定.从表2可知,镀层的显微硬度由20 kHz时的473.3 HV减小到140 kHz的258.8 HV,即随着脉冲频率增加逐渐减小,主要原因是镀层中各相相对数量发生变化,从图1可以观察到CoFe合金镀层中未知相的39.2°峰与bcc相的44.9°峰相对衍射强度随频率提高逐渐增强.采用冶炼法制备的CoFe合金,在热加工薄片过程中,由于材料具有较大的脆性,需要添加贵金属V等元素改善其热加工性能,而用高频脉冲沉积的CoFe合金镀层则具有良好的塑性和韧性,因此具有更高的使用价值.表2 不同脉冲频率下CoFe合金镀层显微硬度Tab.2 Microhardness of CoFe alloy film formed with various pulse frequency频率/kHz硬度/HV 123 4 平均20 523.6 437.4 434.8 497.5 473.380 470.6 533.1 320.6 439.1 440.8140 228.3 226.7 270.1 310.2 258.83 结语实验参数内(20~140 kHz),保持其他工艺参数不变,随着脉冲频率增加,阴极极化作用加强,同时CoFe合金镀层中Fe的原子分数由60.5%下降到49.7%,因而镀层中未知相的39.2°峰与bcc相的44.9°峰相对强度显著增大,结果使镀层晶粒细化,残余压应力由 20 kHz的 590.2 MPa减至140 kHz的366.9 MPa,显微硬度也由473.3 HV减小到258.8 HV.提高脉冲频率,可有效减薄扩散层厚度,使放电离子向电极表面扩散的阻力减小,因而扩散速度加快,阴极电流效率提高,镀层沉积速率增大,膜厚显著增加.从不同频率沉积的CoFe合金薄膜看,因膜层变厚,饱和磁矩由20 kHz的41.77×10-6A◦m2增加到140 kHz的316.1×10-6A◦m2,矫顽力因为受晶粒细化和宏观应力的共同影响,呈非线性变化.采用高频脉冲电流,成功制备了饱和磁感应强度达到2.32 T,而矫顽力却达2626.1 A/m,具有较好软磁性能的CoFe合金薄膜.参考文献:[1]OSAKA T,ASAHI T,KAWAJI J,et al.Development of highperformance magnetic thin film for high-density magnetic recording[J].Electrochemical Acta,2005,50:4576-4585.[2]OSAKA T.Recent development of Magnetic recording head core materials by plating method[J].Electrochemical Acta,1999,44:3885-3890. [3]OSAKA T,SAYAM A J.A challenge of new materials for nextgeneration's magnetic recording[J].Electrochemical Acta,2007,52(8):2884-2890.[4]安茂忠.电镀理论与技术[M].哈尔滨:哈尔滨工业大学出版社,2004.[5]MYUNG N V,PARK D Y,URGILES D E,et al.Electrofo rmed iron and FeCo alloy[J].Electrochemical Acta,2004,49:4397-4404.[6]VILAIN S,EBO THE J.Growth and topology of Co-Fe and Co-Ni alloy electrodeposits studied by atomic force microscopy[J].Materials Science and Engineering C,2001,15:199-201.[7]王子涵,杨滨,铁军,等.脉冲频率对电沉积Ni镀层组织和性能的影响[J].稀有金属材料与工程,2007,36(3):620-622.[8]CHECHENIN N G,du M ARCHIE van VOO RT HU YSEN,DE HOSSON J TH M,et al.Variation of structure and magnetic properties with thickness of thin Co59Fe26Ni15 films[J].Journal of Magnetism and M agnetic Materials,2005,290-291:1539-1542.[9]ZHANG Y H,IVEY D G.Characterization of Co-Fe and Co-Fe-Ni soft magnetic films electrodeposited from citrate-stabilized sulfatebaths[J].Materials Science and Engineering B,2007,140:15-22.[10]ARMYANO S.Crystallographic structure and magnetic properties of electrodeposited cobalt and cobalt alloys[J].ElectrochemicalActa,2000,45:3323-3335.[11]BAI A,HU C C.Iron-cobalt and iron-cobalt-nickel nanowires deposited by means of cyclic voltammetry and pulse-reverseelectroplating[J].Electrochemistry Communications,2003,5:78-82.[12]陈亚.现代实用电镀技术[M].北京:国防工业出版社,2004.[13]ZHANG T Y,CHEN L Q,F U R.Measurements of residual stresses in thin films deposited on silicon wafers by indentation fracture[J].ActaMater,1999,47(14):3869-3878.[14]KOZLOV V M,MAMONTOV E A,PET ROV Y N,et al.Dislocations and internal stresses of electrolytic iron[J].Metalloved,1968,3(26):564-568. [15]田莳.材料物理性能.北京:北京航空航天大学出版社,2007.[16]OSAKA T,YOKOSHIMA T,SHIGA D,et al.A high moment CoFe soft magnetic thin film prepared by electrodeposition[J].Electrochem Solid State,2003,6(4):53-55.。
钌化合物 电化学媒介
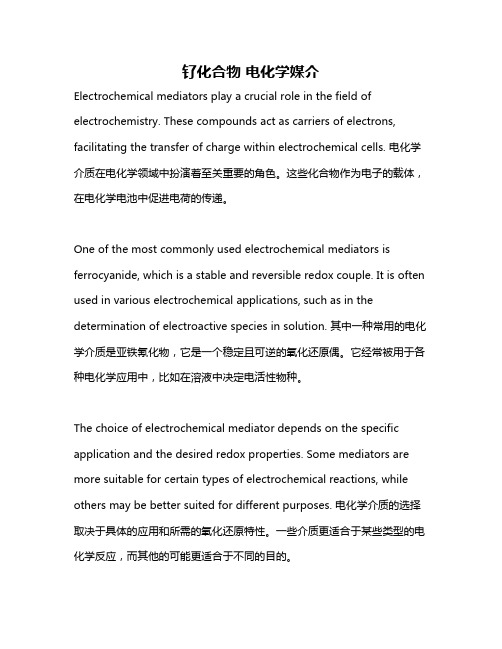
钌化合物电化学媒介Electrochemical mediators play a crucial role in the field of electrochemistry. These compounds act as carriers of electrons, facilitating the transfer of charge within electrochemical cells. 电化学介质在电化学领域中扮演着至关重要的角色。
这些化合物作为电子的载体,在电化学电池中促进电荷的传递。
One of the most commonly used electrochemical mediators is ferrocyanide, which is a stable and reversible redox couple. It is often used in various electrochemical applications, such as in the determination of electroactive species in solution. 其中一种常用的电化学介质是亚铁氰化物,它是一个稳定且可逆的氧化还原偶。
它经常被用于各种电化学应用中,比如在溶液中决定电活性物种。
The choice of electrochemical mediator depends on the specific application and the desired redox properties. Some mediators are more suitable for certain types of electrochemical reactions, while others may be better suited for different purposes. 电化学介质的选择取决于具体的应用和所需的氧化还原特性。
电催化反应的英文
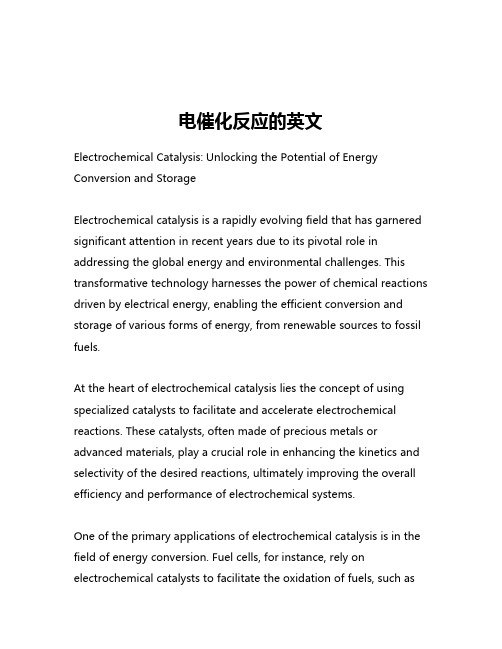
电催化反应的英文Electrochemical Catalysis: Unlocking the Potential of Energy Conversion and StorageElectrochemical catalysis is a rapidly evolving field that has garnered significant attention in recent years due to its pivotal role in addressing the global energy and environmental challenges. This transformative technology harnesses the power of chemical reactions driven by electrical energy, enabling the efficient conversion and storage of various forms of energy, from renewable sources to fossil fuels.At the heart of electrochemical catalysis lies the concept of using specialized catalysts to facilitate and accelerate electrochemical reactions. These catalysts, often made of precious metals or advanced materials, play a crucial role in enhancing the kinetics and selectivity of the desired reactions, ultimately improving the overall efficiency and performance of electrochemical systems.One of the primary applications of electrochemical catalysis is in the field of energy conversion. Fuel cells, for instance, rely on electrochemical catalysts to facilitate the oxidation of fuels, such ashydrogen or methanol, and the reduction of oxygen, generating electricity in a clean and efficient manner. The development of highly active and durable electrocatalysts has been a driving force behind the advancement of fuel cell technology, enabling the widespread adoption of these clean energy devices in various sectors, including transportation, stationary power generation, and portable electronics.Similarly, electrochemical catalysis plays a pivotal role in the storage and conversion of energy from renewable sources. In the case of water electrolysis, catalysts are employed to split water molecules into hydrogen and oxygen, allowing for the storage of energy in the form of hydrogen, which can then be used as a clean fuel or converted back into electricity through fuel cells. This process is particularly important for the integration of renewable energy sources, such as solar and wind, into the energy grid, as it provides a means to store excess energy generated during periods of high production.Moreover, electrochemical catalysis is essential in the developmentof advanced energy storage technologies, such as rechargeable batteries and metal-air batteries. Catalysts are used to enhance the efficiency and durability of the electrochemical reactions that occur during charging and discharging, enabling the storage and retrieval of energy with improved performance and safety.Beyond energy applications, electrochemical catalysis has also found important uses in the fields of environmental remediation and chemical synthesis. In the former, catalysts are employed to facilitate the electrochemical treatment of wastewater, enabling the removal of harmful pollutants and the recovery of valuable resources. In the latter, electrochemical catalysis is used to drive selective chemical transformations, opening up new pathways for the production of various chemicals and pharmaceuticals.The success of electrochemical catalysis is heavily dependent on the development of advanced catalytic materials and the optimization of the catalytic processes. Researchers in academia and industry are continuously exploring new strategies to design and synthesize highly active, selective, and durable catalysts, drawing inspiration from fields such as materials science, nanotechnology, and computational chemistry.One promising approach is the use of nanostructured materials, which offer a large surface area-to-volume ratio and the ability to fine-tune the electronic and structural properties of the catalysts. The incorporation of transition metals, noble metals, and their alloys into these nanostructured materials has led to significant improvements in catalytic performance, with researchers exploring innovative synthesis methods and novel catalyst architectures to further enhance activity and stability.Another area of active research is the development of non-precious metal-based catalysts, which aim to reduce the reliance on scarce and expensive precious metals, such as platinum and iridium. The exploration of earth-abundant elements, including iron, nickel, and cobalt, has yielded promising results, with researchers investigating ways to improve the catalytic activity and durability of these alternative materials.Computational modeling and simulation have also played a crucial role in the advancement of electrochemical catalysis. By coupling advanced computational techniques with experimental data, researchers can gain deeper insights into the underlying mechanisms of electrochemical reactions, enabling the rational design of more efficient and selective catalysts.As the world continues to grapple with the pressing challenges of energy security, environmental sustainability, and resource scarcity, the importance of electrochemical catalysis cannot be overstated. This transformative technology holds the potential to revolutionize the way we produce, store, and utilize energy, while also contributing to the development of more sustainable chemical processes and environmental remediation strategies.Through continued research, innovation, and collaboration amongscientists, engineers, and policymakers, the field of electrochemical catalysis is poised to play a pivotal role in shaping a more sustainable and prosperous future for our planet.。
电化学谱学表征方法的应用与发展
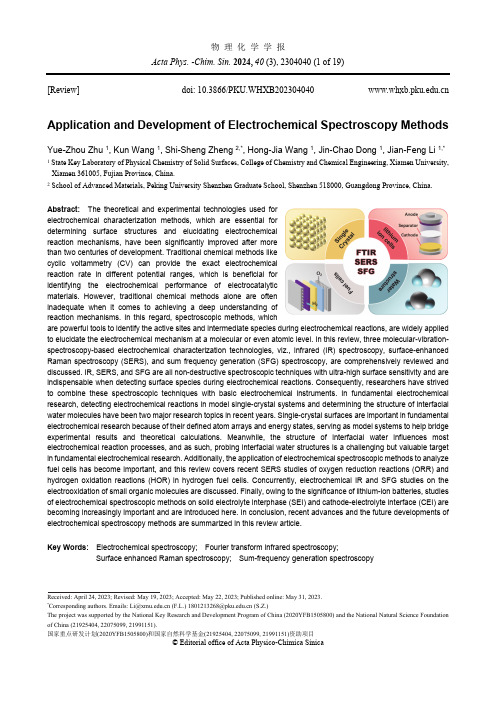
物 理 化 学 学 报Acta Phys. -Chim. Sin. 2024, 40 (3), 2304040 (1 of 19)Received: April 24, 2023; Revised: May 19, 2023; Accepted: May 22, 2023; Published online: May 31, 2023. *Correspondingauthors.Emails:**********.cn(F.L.)******************.cn(S.Z.)The project was supported by the National Key Research and Development Program of China (2020YFB1505800) and the National Natural Science Foundation of China (21925404, 22075099, 21991151).国家重点研发计划(2020YFB1505800)和国家自然科学基金(21925404, 22075099, 21991151)资助项目© Editorial office of Acta Physico-Chimica Sinica[Review] doi: 10.3866/PKU.WHXB202304040 Application and Development of Electrochemical Spectroscopy MethodsYue-Zhou Zhu 1, Kun Wang 1, Shi-Sheng Zheng 2,*, Hong-Jia Wang 1, Jin-Chao Dong 1, Jian-Feng Li 1,*1 State Key Laboratory of Physical Chemistry of Solid Surfaces, College of Chemistry and Chemical Engineering, Xiamen University,Xiamen 361005, Fujian Province, China.2 School of Advanced Materials, Peking University Shenzhen Graduate School, Shenzhen 518000, Guangdong Province, China.Abstract: The theoretical and experimental technologies used for electrochemical characterization methods, which are essential for determining surface structures and elucidating electrochemical reaction mechanisms, have been significantly improved after more than two centuries of development. Traditional chemical methods like cyclic voltammetry (CV) can provide the exact electrochemical reaction rate in different potential ranges, which is beneficial for identifying the electrochemical performance of electrocatalytic materials. However, traditional chemical methods alone are often inadequate when it comes to achieving a deep understanding of reaction mechanisms. In this regard, spectroscopic methods, whichare powerful tools to identify the active sites and intermediate species during electrochemical reactions, are widely applied to elucidate the electrochemical mechanism at a molecular or even atomic level. In this review, three molecular-vibration-spectroscopy-based electrochemical characterization technologies, viz., infrared (IR) spectroscopy, surface-enhanced Raman spectroscopy (SERS), and sum frequency generation (SFG) spectroscopy, are comprehensively reviewed and discussed. IR, SERS, and SFG are all non-destructive spectroscopic techniques with ultra-high surface sensitivity and are indispensable when detecting surface species during electrochemical reactions. Consequently, researchers have strived to combine these spectroscopic techniques with basic electrochemical instruments. In fundamental electrochemical research, detecting electrochemical reactions in model single-crystal systems and determining the structure of interfacial water molecules have been two major research topics in recent years. Single-crystal surfaces are important in fundamental electrochemical research because of their defined atom arrays and energy states, serving as model systems to help bridge experimental results and theoretical calculations. Meanwhile, the structure of interfacial water influences most electrochemical reaction processes, and as such, probing interfacial water structures is a challenging but valuable target in fundamental electrochemical research. Additionally, the application of electrochemical spectroscopic methods to analyze fuel cells has become important, and this review covers recent SERS studies of oxygen reduction reactions (ORR) and hydrogen oxidation reactions (HOR) in hydrogen fuel cells. Concurrently, electrochemical IR and SFG studies on the electrooxidation of small organic molecules are discussed. Finally, owing to the significance of lithium-ion batteries, studies of electrochemical spectroscopic methods on solid electrolyte interphase (SEI) and cathode-electrolyte interface (CEI) are becoming increasingly important and are introduced here. In conclusion, recent advances and the future developments of electrochemical spectroscopy methods are summarized in this review article.Key Words: Electrochemical spectroscopy; Fourier transform infrared spectroscopy;Surface enhanced Raman spectroscopy; Sum-frequency generation spectroscopy电化学谱学表征方法的应用与发展朱越洲1,王琨1,郑世胜2,*,汪弘嘉1,董金超1,李剑锋1,*1厦门大学化学化工学院,固体表面物理化学国家重点实验室,福建 厦门 3610052北京大学深圳研究生院,新材料学院,广东深圳 518000摘要:经历两个多世纪的发展,电化学表征方法的理论和实验研究不断完善,在表界面精细结构表征、电化学反应机理研究等方面起到重要作用。
一种铝电解阳极优化方法,专利
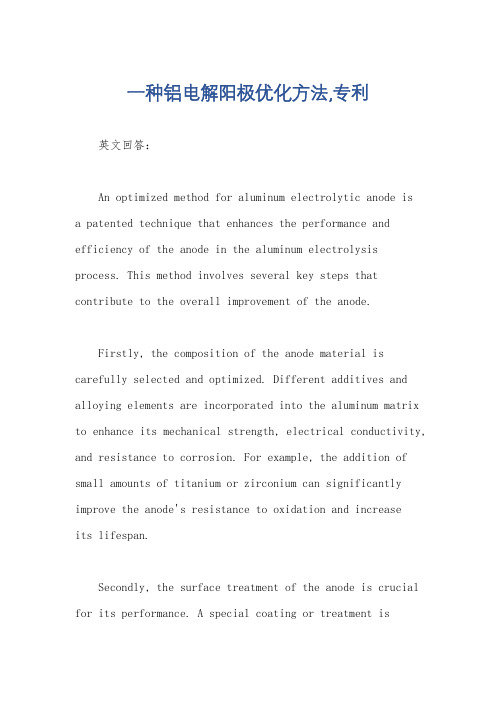
一种铝电解阳极优化方法,专利英文回答:An optimized method for aluminum electrolytic anode isa patented technique that enhances the performance and efficiency of the anode in the aluminum electrolysis process. This method involves several key steps that contribute to the overall improvement of the anode.Firstly, the composition of the anode material is carefully selected and optimized. Different additives and alloying elements are incorporated into the aluminum matrix to enhance its mechanical strength, electrical conductivity, and resistance to corrosion. For example, the addition of small amounts of titanium or zirconium can significantly improve the anode's resistance to oxidation and increaseits lifespan.Secondly, the surface treatment of the anode is crucial for its performance. A special coating or treatment isapplied to the anode surface to enhance its resistance to corrosion and improve its current distribution characteristics. This surface treatment can be a combination of chemical processes, such as anodizing or electroplating, and physical processes, such as shot peening or laser texturing. By modifying the surface properties, the anode can achieve better electrolyte penetration and reduce the formation of unwanted by-products, leading to improved efficiency and longevity.Furthermore, the design of the anode structure plays a significant role in its optimization. The shape, size, and distribution of the anode's current-carrying elements are carefully designed to ensure uniform current distribution and minimize electrical resistance. This can be achieved by using a combination of conductive materials, such as graphite or carbon-based materials, and optimizing the arrangement of these elements within the anode structure. By improving the current distribution, the anode can operate at higher current densities without compromisingits performance.In addition to the material composition, surface treatment, and structural design, the operational parameters of the aluminum electrolysis process are also optimized to maximize the performance of the anode. Factors such as the applied voltage, current density, electrolyte composition, and temperature are carefully controlled to ensure optimal conditions for the anode. By fine-tuning these parameters, the anode can operate at its peak performance and achieve higher energy efficiency.Overall, the optimized method for aluminum electrolytic anode involves a combination of material selection, surface treatment, structural design, and operational parameter optimization. By implementing these steps, the anode's performance, efficiency, and lifespan can be significantly improved, leading to enhanced productivity and cost-effectiveness in the aluminum electrolysis process.中文回答:一种优化的铝电解阳极方法是一种通过改进铝电解过程中阳极的性能和效率的专利技术。
三维电催化处理苯并噻唑反应器结构优化

2017年第36卷第1期 CHEMICAL INDUSTRY AND ENGINEERING PROGRESS·91·化 工 进 展三维电催化处理苯并噻唑反应器结构优化丁杰,宋昭,宋迪慧,刘先树(哈尔滨工业大学市政环境工程学院,黑龙江 哈尔滨 150090)摘要:采用复极性三维电催化反应器降解苯并噻唑,通过考察三维电极反应器的结构参数,从而提高污染物处理效果的同时降低电催化反应体系的能耗。
首先以反应体系的矿化率、矿化电流效率及能耗为评价指标,优化了反应器的电极型式。
然后利用Design-Expert 软件中的Box-Behnken 响应曲面法,以外加电压、电极间距和粒子电极填充比例为自变量,TOC 去除率为响应值,研究了各变量及其相互作用对TOC 去除率的影响,并通过求解回归方程得到多元二次回归方程的预测模型。
结果表明,电极间距和外加电压对TOC 的影响最大,且电极间距和外加电压具有明显的交互性作用。
预测分析最大TOC 去除率为98.18%,相应的最优结构参数为:外加电压9.9V ,电极间距4.2cm ,粒子电极填充比60%,在最优条件下进行试验,实际值与预测值具有良好的一致性,偏差为1.71%。
关键词:电化学;降解;模型;结构参数;苯并噻唑;响应曲面分析法中图分类号:X703.1 文献标志码:A 文章编号:1000–6613(2017)01–0091–09 DOI :10.16085/j.issn.1000-6613.2017.01.012Structural optimization of three-dimensional electrocatalytic reactor forbenzothiazole treatmentDING Jie ,SONG Zhao ,SONG Dihui ,LIU Xianshu(School of Municipal and Environmental Engineering ,Harbin Institute of Technology ,Harbin 150090,Heilongjiang ,China )Abstract :In this study ,bipolar three-dimensional electrocatalytic reactor was applied to benzothiazole degradation and its structural parameters were investigated in order to improve the pollutant removalefficiency and reduce the energy consumption of electrochemistry reactor .Firstly ,the electrode form was improved by comparing the degree of mineralization ,mineralization current efficiency and energy consumption in the reaction system .Then response surface methodology based on Box-Behnken design was successfully applied to analyze the effect of the structural parameters and their interaction on benzothiazole degradation .The effects of three variables ,impressed voltage ,electrode distance and particle electrodes filling ratio upon the total organic carbon removal were evaluated and the prediction model of multivariate quadratic regression equation was acquired .The results showed that electrode distance played the most important role in total organic carbon removal ,followed by impressed voltage and particle electrodes filling ratio ,among which the interaction of electrode distance and the impresses voltage was remarkable .Optimized condition was obtained at 9.9V ,4.2cm and 60% particle electrodes for the reactor .Under the optimal condition ,98.18% of total organic carbon removal was achieved and第一作者及联系人:丁杰(1972—),女,教授,博士生导师,主要从事发酵法生物制氢技术、工业废水处理资源化与综合技术的研究。
电化学脱盐技术及其效果初探

范庆新
FA N Qi ng2x i n1 , Y U Qi2j un2 , CH EN G L i3 , L I Tie2f eng2 , W EI Jiang2x iong2 , ZHA N G Yong2jian4
(11Construction College of Guangdong Technology University , Guangzhou 510643 , Guangdong ,China ;
国外于 20 世纪 80 年代末 ,开始研究并应用电化 学脱盐防锈技术 ,而我国起步相对较晚 ,一些技术诀 窍 ,如电解质溶液种类 、浓度及电流密度 、脱盐时间等 对脱盐效果的影响等尚未完全掌握 。为了加快该技 术尽早在工程实际中的应用 ,本文对电化学脱盐技术 进行了介绍 ,初步探讨了电解质溶液的种类 、浓度及 电流密度等对脱盐效果的影响 。
21 华南理工大学特种功能材料及其制备技术教育部重点实验室 ,广东 广州 510640 ; 31 荆门职业技术学院化工系 ,湖北 荆门 448000 ; 41 广东省建筑科学研究院 ,广东 广州 510500)
[ 摘 要 ] 我国在电化学脱盐防锈技术方面的研究与应用起步较晚 , 一些技术诀窍 , 如电解质溶液种类 、浓度及电 流密度 、脱盐时间等对脱盐效果的影响等尚未完全掌握 。本文对电化学脱盐技术进行了介绍 ,初步探讨 了电解质溶液的种类 、浓度及电流密度等对脱盐效果的影响 。试验结果表明 ,采用 01001mol/ L Li2B4O7 + 饱和 Ca (OH) 2 溶液和 2A/ m2 的电流密度可以取得较好的脱盐效果 。
电化学法英文
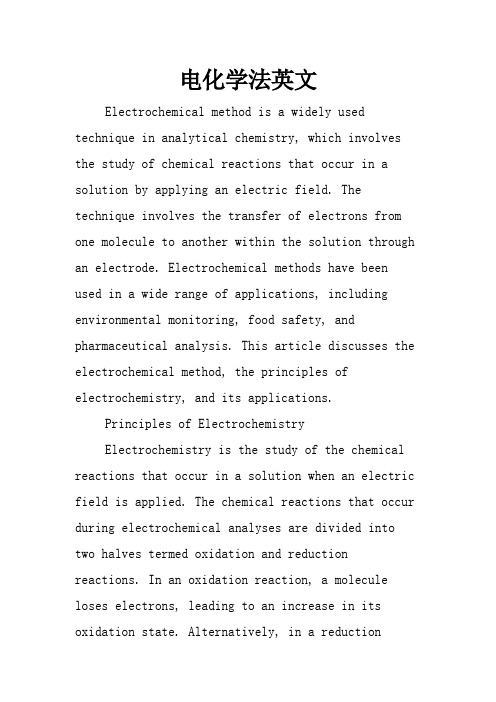
电化学法英文Electrochemical method is a widely used technique in analytical chemistry, which involves the study of chemical reactions that occur in a solution by applying an electric field. The technique involves the transfer of electrons from one molecule to another within the solution through an electrode. Electrochemical methods have been used in a wide range of applications, including environmental monitoring, food safety, and pharmaceutical analysis. This article discusses the electrochemical method, the principles of electrochemistry, and its applications.Principles of ElectrochemistryElectrochemistry is the study of the chemical reactions that occur in a solution when an electric field is applied. The chemical reactions that occur during electrochemical analyses are divided into two halves termed oxidation and reduction reactions. In an oxidation reaction, a molecule loses electrons, leading to an increase in its oxidation state. Alternatively, in a reductionreaction, a molecule gains electrons, leading to a decrease in its oxidation state. These reactions can be described by an equation known as the half-cell reaction equation.During an electrochemical reaction, electrons are transferred between the two electrodes, and a current flows through the solution. This current can be measured using an instrument known as a potentiostat. A potentiostat is a device that applies a voltage to the electrodes, measures the current flowing through the solution, and controls the voltage during the analysis. The voltage that is applied to the electrodes allows the concentration of the target molecule in thesolution to be determined.Electrochemical MethodThe electrochemical method involvestransferring electrons from one molecule to another through an electrode. The molecules thatparticipate in this transfer can be either oxidized or reduced. The electrode used in electrochemical analyses is called an electrode. The electrode isimmersed in the solution, and an electric field is applied to it. This electric field causes the electrons to flow from one molecule to another through the electrode.One of the fundamental techniques used in electrochemical analyses is potentiometry. Potentiometry is the measurement of the potential difference between two electrodes immersed in a solution. This potential difference is proportional to the concentration of the target molecule in the solution. Potentiometry is a powerful technique for the determination of many chemical compounds, including ions and ionizable compounds, such as acids and bases.Another electrochemical technique is amperometry. Amperometry is the measurement of the current flowing through an electrode in response to the electrochemical reaction. This technique is used to investigate the kinetics of the electrochemical reaction and study the chemical reaction under different conditions.ApplicationsElectrochemical method has several applications in analytical chemistry. One of the primary applications of electrochemical methods is the analysis of environmental pollutants. Electrochemical methods can be used to measure the concentration of pollutants in air, water, and soil. These methods are sensitive, inexpensive, and easy to use, making them an attractive choice for environmental monitoring.Electrochemical methods have also been used in food safety analysis. For example, the electrochemical method has been used to detect antibiotics and pesticide residues in food samples. Additionally, electrochemical methods can be usedto analyze food composition and nutritional content, such as the determination of vitamins and minerals in food.Pharmaceutical analysis is another field in which the electrochemical method is used. Electrochemical methods have been used to analyze the concentration of active pharmaceutical ingredients in drugs, which is essential forquality control and regulatory compliance. Additionally, electrochemical methods have been used to study the metabolism of drugs in the body, drug interactions, and drug release in controlled-release formulations.ConclusionElectrochemical method is a powerful analytical technique that measures the electrical propertiesof chemical reactions. The principles of electrochemistry underlie a wide range ofanalytical techniques, including potentiometry and amperometry. Electrochemical methods have many applications in environmental monitoring, food safety, and pharmaceutical analysis. The high sensitivity, low cost, and ease of use of these methods make them an attractive choice for many analytical situations.。
电化学法处理印染废水
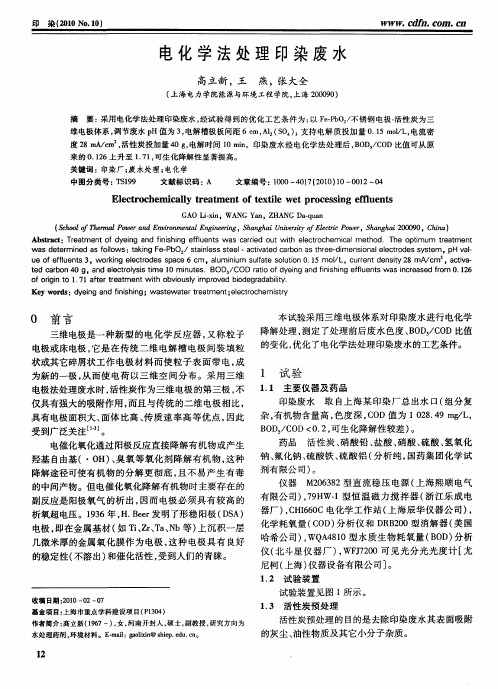
状或其它碎屑状工作 电极材料 而使粒子表面带 电,成
为新 的一极 ,从 而使 电荷 以三 维 空 间 分 布 。采 用 三维 1 试 验
电极法处 理废水 时 ,活性炭 作为 三维 电极 的第 三 极 ,不 1.1 主 要仪器及 药 品
仅具有强大的吸附作用,而且与传统 的二维电极相比,
印染废 水 取 自上 海某 印染 厂 总 出水 口(组分 复
的 中间产物 。但 电催 化氧 化降解 有 机物 时主 要存 在 的
仪器 M206382型 直 流稳 压 电源 (上 海 熙顺 电气
副反应是阳极氧气 的析 出,因而 电极必须具有较高的 有限公 司 ),79HW.1型 恒 温 磁 力搅 拌 器 (浙 江乐 成 电 析氧超 电压 。1936年 ,H.Beer发 明了形 稳 阳极 (DSA) 器 厂 ),CHI660C电化 学工 作 站 (上海 辰华 仪 器公 司 ),
Electrochem ically treatm ent of textile w et processing ef i uents
GAO Li—xin。 W ANG Yan。ZHANG Da·quan
(School of Thermal Power and Environmental Engineering,Shanghai University ofElectr ic Power,Sh a nghai 200090,China)
具有 电极 面积 大 、面体 比高 、传 质 速 率 高 等 优点 ,因此 杂 ,有机 物含量 高 ,色度 深 ,COD值 为 1 028.49 mg/L,
受到广 泛关注 ¨剖。
BeD /COD<0.2,可 生化 降解 性较 差 )。
荧光PCR探针熔解曲线法与微孔板法检测MTB耐药性的临床应用比较

• 132 •中国防痨杂志 2021 年 2 月第 43 卷第 2 期 Chin J Am ituberc ,Fc»bruary 2021,V 〇U 3,N 〇. 2•论著•荧光P C R 探针熔解曲线法与微孔板法检测M T B 耐药性的临床应用比较王佩赵国连雷倩郑丹崔晓利周俊【摘要】目的分析荧光P C K 探针熔解曲线法与微孔板法药物敏感性试验(简称“药敏试验”)检测结核分枝 杆菌(M TB )对抗结核药品耐药性结果的一致性及M T B 基因突变与耐药的相关性,为临床诊疗优化提供参考。
方法搜集2019年1 -12月分离自西安市胸科医院就诊患者并经鉴定确认的343株M T B 临床分离株,菌株均进 行了微孔板法药敏试验和荧光P C R 探针熔解曲线法检测。
以微孔板法药敏试验结果为参照.评价荧光P C R 探针 熔解曲线法检测M T B 对异烟肼、利福平、链霉素、乙胺丁醇、莫西沙星和左氧氟沙星耐药性的检测效能,并分析荧 光P C R 探针培解曲线法检测的M T B 基因突变与微孔板法药敏试验最低抑菌浓度(minimum inhibitory concentre - tio n ,M IC )的相关性。
结果以微孔板法药敏试验结果为参照,荧光P C R 探针熔解曲线法检测M T B 对异烟肼、利福平、链霉素、乙胺丁醇、莫西沙星和左氧氟沙星耐药性的敏感度、特异度、沖《值分别为:96. 20% (76/79)、 95. 28%(242/254)、0. 88:93. 62%(44/47)、94. 58% (279/295)、0. 79; 96. 88% (62/64 )、94. 96% (264/278)、0. 86; 93. 33%(14/15),95. 37% (309/324 ),0. 61;92. 31%(24/26),97. 16%(308/317),0. 80s 91. 18% (31/34) ,99. 35% (307/309)、0. 92。
储能电池有机电极材料改性策略研究进展
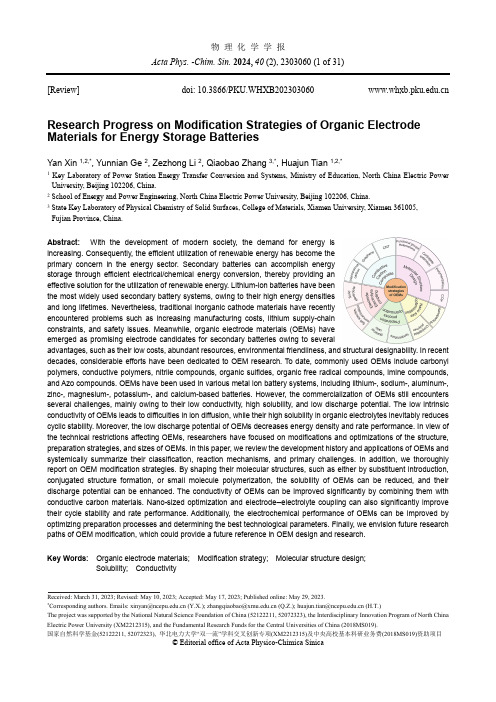
物 理 化 学 学 报Acta Phys. -Chim. Sin. 2024, 40 (2), 2303060 (1 of 31)Received: March 31, 2023; Revised: May 10, 2023; Accepted: May 17, 2023; Published online: May 29, 2023. *Correspondingauthors.Emails:****************.cn(Y.X.);********************.cn(Q.Z.);*********************.cn(H.T.)The project was supported by the National Natural Science Foundation of China (52122211, 52072323), the Interdisciplinary Innovation Program of North China Electric Power University (XM2212315), and the Fundamental Research Funds for the Central Universities of China (2018MS019).国家自然科学基金(52122211, 52072323), 华北电力大学“双一流”学科交叉创新专项(XM2212315)及中央高校基本科研业务费(2018MS019)资助项目© Editorial office of Acta Physico-Chimica Sinica[Review] doi: 10.3866/PKU.WHXB202303060 Research Progress on Modification Strategies of Organic Electrode Materials for Energy Storage BatteriesYan Xin 1,2,*, Yunnian Ge 2, Zezhong Li 2, Qiaobao Zhang 3,*, Huajun Tian 1,2,*1 Key Laboratory of Power Station Energy Transfer Conversion and Systems, Ministry of Education, North China Electric PowerUniversity, Beijing 102206, China.2 School of Energy and Power Engineering, North China Electric Power University, Beijing 102206, China.3 State Key Laboratory of Physical Chemistry of Solid Surfaces, College of Materials, Xiamen University, Xiamen 361005,Fujian Province, China.Abstract: With the development of modern society, the demand for energy is increasing. Consequently, the efficient utilization of renewable energy has become the primary concern in the energy sector. Secondary batteries can accomplish energy storage through efficient electrical/chemical energy conversion, thereby providing an effective solution for the utilization of renewable energy. Lithium-ion batteries have been the most widely used secondary battery systems, owing to their high energy densities and long lifetimes. Nevertheless, traditional inorganic cathode materials have recently encountered problems such as increasing manufacturing costs, lithium supply-chain constraints, and safety issues. Meanwhile, organic electrode materials (OEMs) have emerged as promising electrode candidates for secondary batteries owing to severaladvantages, such as their low costs, abundant resources, environmental friendliness, and structural designability. In recent decades, considerable efforts have been dedicated to OEM research. To date, commonly used OEMs include carbonyl polymers, conductive polymers, nitrile compounds, organic sulfides, organic free radical compounds, imine compounds, and Azo compounds. OEMs have been used in various metal ion battery systems, including lithium-, sodium-, aluminum-, zinc-, magnesium-, potassium-, and calcium-based batteries. However, the commercialization of OEMs still encounters several challenges, mainly owing to their low conductivity, high solubility, and low discharge potential. The low intrinsic conductivity of OEMs leads to difficulties in ion diffusion, while their high solubility in organic electrolytes inevitably reduces cyclic stability. Moreover, the low discharge potential of OEMs decreases energy density and rate performance. In view of the technical restrictions affecting OEMs, researchers have focused on modifications and optimizations of the structure, preparation strategies, and sizes of OEMs. In this paper, we review the development history and applications of OEMs and systemically summarize their classification, reaction mechanisms, and primary challenges. In addition, we thoroughly report on OEM modification strategies. By shaping their molecular structures, such as either by substituent introduction, conjugated structure formation, or small molecule polymerization, the solubility of OEMs can be reduced, and their discharge potential can be enhanced. The conductivity of OEMs can be improved significantly by combining them with conductive carbon materials. Nano-sized optimization and electrode –electrolyte coupling can also significantly improve their cycle stability and rate performance. Additionally, the electrochemical performance of OEMs can be improved by optimizing preparation processes and determining the best technological parameters. Finally, we envision future research paths of OEM modification, which could provide a future reference in OEM design and research. Key Words: Organic electrode materials; Modification strategy; Molecular structure design;Solubility; Conductivity储能电池有机电极材料改性策略研究进展辛燕1,2,*,葛运年2,李泽中2,张桥保3,*,田华军1,2,*1华北电力大学,电站能量传递转化与系统教育部重点实验室,北京 1022062华北电力大学能源动力与机械工程学院,北京 1022063厦门大学材料学院,固体表面物理化学国家重点实验室,福建厦门 361005摘要:有机电极材料因其成本低、资源丰富、环境友好、可设计性等优势,成为具有发展潜力的二次电池候选电极材料。
用于SO2去极化电解制氢的铂基催化剂

化工进展Chemical Industry and Engineering Progress2023 年第 42 卷第 S1 期用于SO 2去极化电解制氢的铂基催化剂谢璐垚,陈崧哲,王来军,张平(清华大学核能与新能源技术研究院,北京 100084)摘要:综述了铂基SO 2去极化电解(SDE )阳极催化剂的研究进展。
SDE 阳极反应条件苛刻,铂基催化剂因具备良好的导电性、抗腐蚀性,并能够有效抵抗H 2S 等硫物质的毒化,成为SDE 阳极催化剂的首选。
通过引入Al 、Cr 、Ni 等非贵金属元素,可有效提高铂基催化剂性能并减少Pt 的用量。
在载体方面,综述和讨论了活性炭、石墨、炭黑、石墨烯以及SiC/TiC 等对铂基催化剂性能的影响,此外分析了催化剂制备工艺对催化剂结构参数和性能的影响。
尽管已经取得了很多研究成果,但当前对铂基SDE 阳极催化剂的长期稳定性、多金属催化剂各金属元素间的相互作用等方面的研究尚较少,进一步优化催化剂设计、加强载体筛选及其改性,开发新的制备工艺,提高Pt 利用率及催化剂的活性和稳定性,是未来相关研究的关键所在。
关键词:制氢;混合硫循环;二氧化硫去极化电解;铂基催化剂中图分类号:TQ15 文献标志码:A 文章编号:1000-6613(2023)S1-0299-11Platinum-based catalysts for SO 2 depolarized electrolysisXIE Luyao ,CHEN Songzhe ,WANG Laijun ,ZHANG Ping(Institute of Nuclear and New Energy Technology, Tsinghua University, Beijing 100084, China)Abstract: The research progress of platinum-based SO 2 depolarized electrolysis (SDE) anode catalysts is reviewed in this paper. Because of the outstanding electrical conductivity, corrosion resistance, and effective resistance to H 2S and other sulfur-containing poisonings, platinum-based catalysts are considered as the best choice for SDE anode. By introducing non-precious metal such as Al, Cr and Ni, the performance of platinum-based catalysts can be effectively improved and the amount of Pt can be reduced. In terms of the support, the effects of activated carbon, graphite, carbon black, graphene and SiC/TiC on the properties were reviewed and discussed. In addition, the effects of catalyst preparation technology on the structural parameters and performance of catalysts were also discussed. Although many research results have been achieved, there are still insufficient studies on the long-term stability of platinum-based SDE anode catalysts and the interactions among metal elements in polymetallic catalysts. Further optimization of catalyst design and carrier screening/modification, development of new preparation processes, improvement of Pt utilization and catalyst activity and stability, are the keys of future research.Keywords: hydrogen production; hybrid sulfur cycle; SO 2 depolarized electrolysis; platinum based catalyst综述与专论DOI :10.16085/j.issn.1000-6613.2023-1169收稿日期:2023-07-10;修改稿日期:2023-09-12。
Design and optimization of electrochemical microreactors for continuous electrosynthesis

ORIGINAL PAPERDesign and optimization of electrochemical microreactors for continuous electrosynthesisC.Renault •J.Roche •M.R.Ciumag •T.Tzedakis •S.Colin •K.Serrano •O.Reynes •C.Andre´-Barre `s •P.Winterton Received:4January 2012/Accepted:3July 2012/Published online:26July 2012ÓSpringer Science+Business Media B.V.2012Abstract The study focuses on the design and construction,as well as the theoretical and experimental optimization of electrochemical filter press microreactors for the electrosyn-thesis of molecules with a high added value.The main char-acteristics of these devices are firstly a high-specific electrochemical area to increase conversion and selectivity,and secondly the shape and size of the microchannels designed for a uniform residence time distribution of the fluid.A heat exchanger is integrated into the microstructured electrode to rapidly remove (or supply)the heat required in exo-or endothermic reactions.The microreactors designed are used to perform-specific electrosynthesis reactions such as ther-modynamically unfavorable reactions (continuous NADH regeneration),or reactions with high enthalpy changes.Keywords Electrochemistry ÁMicroreactors ÁElectrosynthesis ÁFluorination ÁNADH regeneration ÁOptimization ÁSimulation ÁPOISEUILLE ÁPressure drop List of symbols C or c Concentration (mol L -1)C p Heat capacity of the coolant EG (2,400J Ákg -1ÁK -1)C p,j Specific heat of each reactant or product jD Diffusion coefficient (m 2s -1)DG Deoxy-D -glucose DME Dimethoxyethane E Potential (V)E elect.E exch.Electrical and exchanged energy,respectively EG Ethylene glycole blThickness of the metallic fin of the heat exchanger (m)Et 3N Á3HF /TEAHF Triethylamine trihydrofluorideF Faraday constant (96,485C mol -1)F °Molar flux at the input and the output of the microreactorFAD/FADH 2Flavin redox mediator (oxidized and reduced forms)FDH Formate dehydrogenase enzyme FDG Fluoro-deoxy-D -glucose g Acceleration due to gravity9.80665m s-2h Convective exchange coefficient of the coolant (W m -2K -1)i Current density (A m -2)k Mass transfer coefficient (m s -1)KEquilibrium constantC.RenaultLaboratoire de Ge´nie Chimique,Universite ´de Toulouse,INP-ENSIACET,31030Toulouse,FranceJ.Roche ÁM.R.Ciumag ÁT.Tzedakis (&)ÁK.Serrano ÁO.ReynesLaboratoire de Ge´nie Chimique,Universite ´de Toulouse,UPS,118,route de Narbonne,31062Toulouse,France e-mail:tzedakis@chimie.ups-tlse.frS.ColinUniversite´de Toulouse;INSA,UPS,Mines Albi,ISAE;ICA (Institut Cle´ment Ader),135Av.de Rangueil,31077Toulouse,FranceC.Andre´-Barre `s Laboratoire de Synthe`se et Physicochimie des mole ´cules d’inte ´re ˆt biologique,Universite ´de Toulouse,UPS,118,route de Narbonne,31062Toulouse,FranceP.WintertonDe´partement Langues &Gestion,Universite ´de Toulouse,UPS,118,route de Narbonne,31062Toulouse,FranceJ Appl Electrochem (2012)42:667–677DOI 10.1007/s10800-012-0445-xk cat Rate of the enzyme catalyzedreaction(s-1)K m,j Michaelis–Menten constants(L-1mol-1)L Length of the considered‘‘reactive’’channel or microchannel segment(m) L bl Length of the metallicfin of the heatexchanger(m)n Electron numbern l Number of microchannels on theplateNAD?/NADH Nicotinamide dinucleotide pyridiniccofactor(oxidized and reducedforms)Nu Nusselt number(Nu=h L bl/k)p Pressure(Pa)p exch Overall perimeter of thefin section(m)Po Poiseuille numberQ Charge(F mol-1)Q v Volumetricflow rate(m3s-1)R Universal gas constant(8.31J mol-1K-1)I Current(A)r j Rate of homogeneous reactions(mol m-3s-1)r v R Rate of chemical reaction(mol m-3s-1)S Cross-section area of theflowchannel(m2)S a Electrode active surface area(m2)S exch Total surface area of all thefins(m2) t Time(s)T Absolute temperature(K)TBAP Bu4NClO4u EG Coolant velocity(0.02mÁs-1)V EC Electrolytic compartment volume(m3)X Conversion of the limiting reagent x,y,z Axis coordinateD f Hj Standard formation enthalpy of the species j(J mol-1)D R H°Standard reaction enthalpy(J mol-1) D R G°Standard reaction-free enthalpy(J mol-1)D P Pressure drop(Pa)D V Cell voltage(V)h Opening angle of the distributingand/or collecting channels(°)k Coolant thermal conductivity(W m-1K-1)l Dynamic viscosity Pa s)m j Stoichiometric coefficientq Specific gravity of the coolant(1,109kgÁm-3)s Residence time in the reactor(s)t!Fluid velocity(m s-1)/Heatflux(J s-1)1IntroductionThis study deals with the design and optimization(theo-retical and experimental)of electrochemical microreactors for the electrosynthesis of various types of molecules with a high-added value.The microreactor is expected to operate continuously,under conditions allowing minimi-zation of both costs and risks of the isothermal synthetic processes.A high-specific area of the electrochemically active surface increases conversion and selectivity.2Microreactor design and construction2.1Microreactor designThis part of the study concerns the design of microstruc-tured electrodes and the incorporated heat exchanger.Their shape and size should allow a uniform residence time distribution for efficient and selective electrochemical reactions.Square or semi-circular microchannel cross-sections yield a specific surface area of at least200cm-1.2.1.1Optimal geometry of the microreactorTo carry out preparative electrosynthesis of valuable adducts using microreactors able to operate under volu-metricflows in the range of1\Q v(cm3/min)\10,an appropriate hydraulic diameter of the microchannels is a few tens of l m.Consequently the influence of electroki-netic or electrocapillarity phenomena can be ignored in the calculations.Poiseuille’s law is used to evaluate the pres-sure drop between the various parts of the inlet and outlet channels as well as through the microchannels[1–4].The electrolytic compartment is lined by microgrooves cut into the solid electrode.Various shapes are possible for the electrodes,the microchannels,and the distributing/col-lecting channels(Fig.1).Both the length and the width of the microchannels,as well as the openness of the distrib-uting and collecting channels are parameters that must be optimized to improve the efficiency of the microdevice.To propose a microdevice with a specific surface area (for the electrochemical reaction)in the range of200–300cm -1,we chose to cut between 30and 40microchannels/cm.To avoid dead volumes in the electrolytic compartment,the overall dimensions of the distributing and collecting channels must be minimized.In addition the influ-ence of their openness on the residence-time distribution of the flow constitutes the main goal of a study recently published [2],and of which the mains lines are summarized below:An approached simulation (performed using Scilab software):the distributing and collecting channels are assumed to be a sequence of short segments of length L and with a rectangular cross-section.For each segment Poiseuille’s law (1)is applied to evaluate the pressure drop D P (related to the corresponding flow Q v ).D P ¼Po 2l l Q v =SD 2hð1ÞThe Poiseuille number for a rectangular cross-section can be calculated [5]from the following polynomial Eq.(2):Po R ¼241À1:3553r ÃR þ1:9467r Ã2R þ1:7012r Ã3RÀÀ0:9564r Ã4R À0:2537r Ã5R Áð2Þwhere 0\r R *\1is the aspect ratio of the rectangular section defined by the depth:width ratio.The main assumption for this simplified approach is that the outlet pressure of each segment of the distributing channel is assumed to be the same to the pressure at the inlet of the corresponding microchannel (Fig.2a);in the same way,the outlet pressure of each microchannel is assumed to be the same to the pressure at the inlet of the corresponding segment of the collecting channel (Fig.2a).For instance,in the case of an electrode with ten mi-crochannels,the system to solve contains 32equations (11for the distributing channel,10for the microchannels,and 11for the collecting channel).The results give the optimal opening angle h of the distributing and collecting channels (Fig.2b)to allow uniform distribution of flow between the microchannels,(Fig.3).A rigorous treatment was performed by CFD calcula-tions (Fluent),solving the Navier–Stokes equations,and the results [2]lead to ‘‘accurate’’velocity and pressure drop profiles within the microstructured electrode.This rigorous approach requires long calculation times,so a more rapid hybrid model was prepared:the distributing and collecting channels are simulated by CFD calculation,while the reaction microchannels are simulated bytheFig.1Various possible shapes for microstructured electrodes.a Standard shape including distributing,collecting channels,and reaction microchannels.b Longer reaction microchannels.c Largerdistributing and collecting channels.d Larger reaction microchannels.e Variation of the openness of distributing and collectingchannelsFig.2Schematic representation of the microstructured electrode;chosen example:10reaction microchannels;Length:5mm for ‘‘micro-‘‘,‘‘collecting-’’,and ‘‘distributing-’’channels;depth:50l m for ‘‘micro-’’,‘‘collecting-’’,and ‘‘distributing-’’channels;width of microchannels:250l m;width for collecting and distributing chan-nels 1mm.a A possible non-optimized geometry.b Optimized geometry by variation of the opening angle h of the distributing and collecting channels [2]analytical approach,where the phenomena are well known.This hybrid approach,allowing refinement of the mesh in the complex sections of the flow,provides more accurate data than the simple CFD approach,for the same compu-tational effort.The agreement between analytical data and results from hybrid simulation is good,with a mean devi-ation of the flow rate of less than 4%.2.1.2Heat exchanger associated with microstructuredelectrode A steady-state thermal balance was performed to define the area required for a heater to provide rapid removal (or supply)of heat for exo/endo thermic reactions;the main idea is to facilitate heat transfer between the reaction area and a coolant.Two kinds of electrodes are examined:(a)Platinum-covered microstructured silicon wafers.In this case,because of the fragility of the silicon wafer,a Teflon block was used as a backing support as well as for the tank containing the heat-exchange liquid.No specific estimation of the performance of this heat exchanger was performed.(b)Microstructured flat metal fins (e.g.,platinum andgold)soldered to a copper block with a ‘‘high area’’heat exchanger on the back.The heat exchanger used was composed of a series of long thin rectangular fins dipped into a tank containing a flow of coolant (Fig.4).The fins are parallel to the coolant flow,and perpendicular to the reaction mixture flow.A thermal balance taking into account the fluorination reaction enthalpy,as well as the electrochemical irrever-sibilities (ohmic drop),is required to estimate the exchange area,i.e.,the number and the size of fins,as a func-tion of the operating parameters (e.g.,volumetric flow,conversion,reagent concentration,coolant temperature,etc.),in order to operate,at steady state,under isothermal conditions within the electrolytic compartment.The target reaction chosen in order to design and man-ufacture the heat exchanger (determination of the exchange area)was the fluorination of 2-deoxy-D -glucose (DG)leading to 2-fluoro-2-deoxy-D -glucose (FDG).DG þF 2!FDG þHFð3ÞTaking into account the bond energies of the molecules involved,the formation enthalpies of various atoms in the gas phase,and finally using thermodynamic cycles,it is possible to access the reaction enthalpy [3],by calculation offormation enthalpies of all reagents (D f HDG C 6H 12O 5=-746.9kJ Ámol -1;D f HFDG C 6H 11FO 5=-956.59kJ Ámol -1;D f H HF =-267.6kJ Ámol -1).The result (the enthalpy change for the fluorination reaction of the DG:D R H °=-477.2kJ mol -1)indicates an exothermic reaction.For an ‘‘open’’,assumed plug-flow,microreactor oper-ating at steady state,the overall energy balance,based on the first law of thermodynamics,can be written as follows:Output enthalpy Àinput enthalpy¼mechanical energy þelectrical energy þthermal energyð4ÞBy introducing the converted flux F c (mol Ás -1)the energy balance can be written as follows:F c output enthalpy Àinput enthalpy ðÞ¼mechanical power þelectrical power þthermal powerð4a ÞBy expressing the enthalpy using Kirchhoff’s law,and operating under isothermal conditions,the first term of this equation can be simplified to the reaction enthalpy (corresponding to the converted flux of the limitingreactant).Fig.3Influence of the opening angle h of distributing and collecting channels on the volume flow rate distribution within the microstruc-tured electrode (described in Fig.2).a h =0°.b h =10.3°.c h =10.6°.d h =10.9°XproductsÀreactantsm j C p;j d T¼0ð5Þfor reactions under‘‘isothermal conditions’’.Consequently,in the absence of mechanical energy and assuming no influence of the pressure in the reaction enthalpy change,the overall energy balance(expressed in J s-1or W)can be written:F c D R H¼electrical powerþexchanged heat flux)Q v D R H C0X¼i S a D VþE exch:ð6Þwhere D V is the cell voltage,i the current density,and S a the electrode area;X is the conversion during operating time(here the residence time s);Q v is the volumetricflow rate and C0the initial molar concentration of the reactant.Assuming that the microreactor operates in galvano-static‘‘optimal’’conditions(i.e.,I applied=I limiting),the conversion rate X can be expressed according to:X¼1Àexp fÀS a k=Q v gð7Þwhere k is the mass transfer coefficient.To evaluate the area required for the heat exchanger,it is necessary to establish the temperature profile between the bottom of thefin(z=0)and the coolant;this profile gives access to the exchanged energy E exch.To do this, some assumptions will be made:–The reaction mixture must remain within the micro-channels at constant temperature.–The maximum temperature difference allowed between the inlet and the outlet of the coolant is10°C.–Copper was used as material to construct the heat exchanger because of its good thermal conductivity;consequently we assume that the heatflux density received by the copper block is the same for any position on axes x and y.Consequently the temperature of the block is equal in any position(x,y,z=0)and noted T wa.Thermal transfer limitations are due to the metalfins.–The total area of thefins has to be*95%of the area of the copper block bottom in contact with the coolant.Taking into account the heatflux(/z(Z))across section z,as well as the heat exchanged by convection(d/c),the thermal balance for segment d z of thefin(Fig.4)can be written according to Eq.(8).k S exchd Td zzþd zÀd Td zz&'¼hp exch d z T zÀT extðÞ)d2Td z2Àhp exchk S exchTÀT extðÞ¼0ð8ÞIn the case of rectangularfins with constant cross-section and heat transfer at the end of thefins,the boundary conditions can be written as:T z=0=T wa and Àk S exch d T dzz¼L bl¼hS exch T z¼LblÀT extðÞSolving(8)provides the temperature profile T(Eq.9): T zÀT extðÞT waÀT extðÞ¼cosh x L blÀzðÞðÞþhxksinh x L blÀzðÞðÞcosh x L blðÞþh sinh x L blðÞð9Þwhere S exch is the total area of allfins,T,T wa,and T ext the local temperature,blade wall temperature,and temperature far from thefin,p exch the overall perimeter of thefin sec-tion,h the convective exchange coefficient of the coolant (here507W m-2K-1),k the thermal conductivity of the coolant,x:ffiffiffiffiffiffiffiffiffihp exchk S exchqThe heatflux/th=-k S exch(d T/d z)z=0,i.e.,the E exch., exchanged between the coolant and thefin(of which the length is L bl)can be written according to Eq.(10):/th¼xk S exch T waÀT extðÞtanh x L blðÞtanh x L blðÞþhxk1þhxktanh x L blðÞð10ÞTaking into account the conditions of these experiments:The exchange convective coefficient h of the coolant(ethylene glycol EG)was estimated using Nusselt’s correlation:Nu¼hÃL bl=k¼constant x Re0:5Pr0:33¼0:664ðq L bl u EG=lÞ0:5ðC p l=kÞ0:33ð11Þwhere q the specific gravity(1109kgÁm-3),l the dynamic viscosity(0.02Pa s),C p the heat capacity(2.4kJÁkg-1ÁK-1), and u EG the coolant velocity(0.02mÁs-1).In these conditions,and considering L bl=0.1m,the Nusselt’s value was estimated to be39.For long(L bl C0.1m)thin(e bl B1mm)metalfins,the heatflux exchanged between the coolant and thefin can be simplified as follows:/th¼E exch¼xk S exch T waÀT extðÞtanh x L blðÞ:ð12ÞThis equation is used in the energy balance(6)to lead to thefinal energy balance Eq.(6b)allowing evaluation of the heat exchanger area.0¼I S a D VÀQ v D R H C0ð1ÀexpðÀS a k=Q vÞÞþxk S exchðtanhðxkÞÞT waÀT extðÞ:ð6bÞThe cell voltage term(D V),corresponds to irreversibilities(overvoltages)and ohmic drops;its value, was estimated by:–Current–potential curves plotted using a rotating disk electrode as working electrode,under various condi-tions.The main factor varied was the concentration of fluorination agent[3].–Resistance measurements of the solution and the separator between the anodic and cathodic compart-ments (the overall resistance for the microreactor estimated at 223X ).Typical values of the electrochemical term (I D V )were between 0.5and 2W for a geometrical area of the elec-trode of about 10cm 2and relatively high reagent con-centrations (from 0.5to 1mol l -1).The value of the chemical term (changes in enthalpy)was 20–30W for similar reagent concentrations,volu-metric flows lower than 5cm 3min -1,and high conversion values (X [85%).Consequently,for electrochemical microreactors with an electrode geometrical area of *10cm 2,the corresponding power to be removed by the coolant is about 20–30W and the surface area of the heat exchanger (S exch )has to be at least 5cm 2.Note that this value covers uncertainties (one to two times the expected value)due to various approximations in the calculations.2.2Microreactor constructionTwo different ways were used in this study to prepare the microstructured electrodes.First,soft lithography was used to groove a silicon wafer (Fig.5a)with 150rectangular-section microchannels with an opening angle for inlet/outlet channels of h =3.7°.A *5l m platinum layer was deposited by PVD on the microstructured wafer.However,poor adhesion of the platinum on the silicon/chromium significantly reduces the practical utility of the systems as electrosynthesis anodes.Micro/nano-scratch (micro/nano-indentor)device,with a homemade needle,was used to create semi-ellipsoidal microgrooves on a gold and platinum plate.The excess metal accumulated at the microchannel sides was removed by polishing,and a satisfactory shape of microchannels was obtained (Fig.5b).The main idea was to allow easy heat transfer between the reaction area and the coolant.When the electrodes were microstructured metal plates,the electrode (platinum,gold)was soldered to a copper block with a heat exchanger on the other side.The heat exchanger was composed of sev-eral long thin metal fins dipped in a tank in which coolant flowed,as indicated in Fig.5c,d.The fins were parallel to the coolant flow,and perpendicular to the reaction mixture flow.The microchannel area was not perfectly smooth;nevertheless,the presence of microscopic holes/roughness (depth of holes/depth of microchannels \3%)within the microchannel is beneficial to mass transfer.On the final electrode (Fig.5d)there were 152semi-ellipsoidal microchannels,with h =4.8°and specific area S a /V EC *210cm -1.The micromechanically prepared heat exchanger (Fig.5c)with its thirteen fins had a global area of 52cm 2(ten times the required area for the currentapplication).Fig.5Pictures of the microstructured electrodes and heater.a Microstructured silicon wafer,covered with platinum and contain-ing n l =150microchannels.b An ‘‘increasing size’’microgroove,made using a microindentor.c Heat exchanger (13fins,total surface area of 52cm 2).d Integrated block contains the copper heat exchanger and the microgooved platinum electrode (152semi-ellipsoidal microchannels cross-section:length:35mm;depth:50l m;width:150l m;microchannels overall geometrical area =n l *p *length*{(depth)2?(width)2}0.5/2=13.2cm 2;microchannels overall volume =n l *p *length*(depth/2)*(width/2)/2=0.062cm 3;the width of the distributing/collecting channels decrease from the inlet (*3mm)to the outlet (*0mm);S a /V EC *210cm -1).e Preparative electrolysis set-upThis fin area would allow a very large range of applications for instance solutions with higher concentrations and flow rates,as well as more exothermic reactions.3Microreactor for electrosynthesis:experimental results The microstructured electrodes/heat exchanger block (Fig.5d)was included in the microreactor (Fig.5e)to perform electrosynthesis in two different ways:organic media for anodic fluorination reactions and aqueous media for enzymatic and ‘‘electrochemically assisted’’chiral syntheses.3.1Anodic fluorination in the microreactor:fluorination of deoxy glucose in dimethoxy ethane and acetonitrile The target molecule,deoxy-glucose,was studied in two solvents:acetonitrile and dimethoxyethane.The goal here was to carry out the reaction without risks (gaseous fluo-rine),in one electrochemical step instead of several chemical synthesis steps [requiring protection of the OH groups before fluorination in a second-order nucleophilic substitution,as described by reaction (13)][6].Moreover,the use of the heat exchanger allows fast heat transfer and better chemical selectivity.Various fluorination agents (e.g.,(CH 3CH 2)3N*3HF noted TEAHF,CsF,HF)were tested to carry out this reaction.The current–potential curves (curve 1,Fig.6),obtained with the most appropriate fluorination agent,TEAHF in acetonitrile [7],shows two waves.The first (0.5–1.5V)is due to the oxidation of the adsorbed TEAHF on the electrode and the second (1.5–2.7V)corresponds to the oxidation of free TEAHF in solution [3,7].Curve 2indicates low current for DG oxidation (signal from 1.2to 1.8V).Because of the low solubility of this substrate in acetonitrile,we were unable to rigorously compare curves 1and 2,and could not conclude on the electrochemical activity of DG which seems very low.Nevertheless,it seems obvious that the fluorination agent TEAHF was oxidized before the deoxy-glucose.Preparative electrolysis was carried out under various conditions to perform fluorination of DG in DME,CH 3CN and a mixture of the two.Fluorination was fol-lowed using 19F NMR spectroscopy.The results,(19F NMR)show that both the substrate (DG)and the solvent (DME)were fluorinated.This was surprising as DME seemed difficult to fluorinate.However,Hou and Fuchi-gami [8]reported fluorination of DME,and proposed a reaction scheme (Reaction 14),using F -supplied by various forms of TEAHF (Et 3N*3HF,Et 3N*5HF Et 4N*3HF Et 4N*5HF)in acetonitrile;this reaction could lead to twoisomers.ð13ÞH 3CO +O CH 3H 2CO +O CH 33CO +OCH 3H 3COOCH +F --FH 2CO OCH 3H 3CO OCH 3F --++2a3a.ð14ÞThe results of this study are listed in Table1.NMR yielded four peaks at-136,-137,-151,and-154ppm on the horizontal axis.The peak at-111ppm was used as reference(sodiumfluorobenzoate)[3].Fine analysis of the NMR spectra did not indicate compounds containing two fluorides on the same pound2a was found to be the majority product becausefluorination of the methyl group is easier thanfluorination of the methylene function. Difluorinated products such as FH2COCH2CH2OCH2F (2a0)and H3COCHFCH2OCH2F(3a0)were also obtained.For high values of the charge Q(runs1–3),decreasing the temperature caused the selectivity for2a and2a0to increase. Similar results were obtained for lower charges(runs4–6), Table2.Runs1–7were carried-out with2-deoxy-D-glucose, while runs10–70were performed without.The expectedfluorination of the DG(DG is indicated as:–CHOH–CH2–CHOH–)can be written as follows:ÀCHOHÀCH2ÀCHOHÀþFÀ!Hþþ2eÀþÀCHOHÀCHFÀCHOHÀ1ÀFDGðÞand=orÀCHOHÀCH2ÀCFOHÀ2ÀFDGðÞð15ÞNMR results for runs1and2showed the presence of a peak at-184ppm,close to the peak of commercial2-FDG (-200ppm).This means that the DG wasfluorinated,but not at the expected position(2-FDG).We were not able to identify the exact formula of the reaction product,and CsF was not retained asfluorinating agent.Similar behavior was observed for runs5and6,where the peak at-192ppm corresponded to the expected2-FDG.Note that a temperature decrease has a negative effect on the2-DG fluorination;indeed,runs3and7carried out at-10°C did not indicate the presence of2-FDG.This result is surprising because numerous authors indicate the exothermicity of the fluorination reaction,and the opposite behavior was expected.On the other hand,NMR analysis showed the presence of peaks from-135to-170ppm,which were attributed to the CsF,or to adducts of the DMEfluorination, as well as Et3N–3HF decomposition adducts.Note that for all experiments the ratio‘‘supplied amount of charge:required amount of charge’’was higher than1and reached18.5in electrolyses5–7.To sum up,the microreactor allowedfluorination of 2-deoxy-D-glucose in one step although the overall process needs optimization,mainlyfluorinating agent separation and2-fluoro-2-deoxy-D-glucose purification.The electrical consumption,even though in excess,remained low,in comparison with the cost of the adduct produced.3.2NADH:continuous electroenzymatic regeneration Many dehydrogenation reactions involve the use of the py-ridinic cofactor NADH for the synthesis of optically active compounds.Because of the high cost of NADH(70€/g),its regeneration constitutes an economic challenge.As far as we know,no industrial process allowing continuous in situ regeneration of this cofactor exists.Many electrochemical studies have reported indirect electrochemical synthesis of NADH using various redox mediators[9,10].Here,the electrochemical microreactor we designed was used in theTable1Preparative electrolyses for dimethoxyethanefluorination within microreactorRun DME(mol l-1)Et3N–3HF(mol l-1)I/mA t/min Q/F mol-1T/°C Yield(%)of thefluorinated products/d(ppm)Monofluorinated2a/-151Monofluorinated3a/-136Difluorinated2a0/-154Difluorinated3a0/-13710.0120.125607.77151810211 20.0050.025111207.14-5275300 3-10275290 40.0110.021160 3.731510.2 2.5 6.40 5510.9 2.37.80 6-510.5 2.38.70Anode and cathode are made of platinum;solvent CH3CN.Anolyteflow=catholyteflow=0.2cm3min-1。
电化学脱合金的英文

电化学脱合金的英文Electrochemical Dealloying: Principles, Applications, and Challenges.Introduction.Electrochemical dealloying is a process that involves the selective removal of one or more constituent metalsfrom a multicomponent metallic alloy by electrochemical means. This process, often referred to as "dealuminization" in the context of aluminum-based alloys, has found widespread applications in materials science, nanotechnology, and energy conversion and storage systems. The primary advantage of electrochemical dealloying lies in its ability to create nanostructured materials with unique physical and chemical properties, such as high surface area, porosity, and conductivity.Principles of Electrochemical Dealloying.The electrochemical dealloying process occurs when an alloy is immersed in an electrolyte solution and apotential is applied between the alloy and a counter-electrode. The applied potential drives the electrochemical reactions at the alloy surface, resulting in thedissolution of one or more constituent metals. The dissolution rate of each metal depends on its electrochemical properties, such as the redox potential and electrochemical activity in the given electrolyte.During the dealloying process, the alloy is typically the anode, and the counter-electrode is the cathode. The anode is connected to the positive terminal of the power source, while the cathode is connected to the negative terminal. When the potential is applied, the alloy begins to dissolve, and the dissolved metal ions migrate towards the cathode. At the cathode, the metal ions are reduced and deposited on the surface, forming a new metal layer.The rate of metal dissolution during electrochemical dealloying is controlled by several factors, including the electrolyte composition, applied potential, temperature,and alloy composition. By optimizing these parameters, researchers can precisely control the morphology, porosity, and composition of the resulting nanostructured materials.Applications of Electrochemical Dealloying.Electrochemical dealloying has found numerous applications in materials science and engineering. Some of the key applications are discussed below:1. Nanoporous Metals: Electrochemical dealloying is widely used to create nanoporous metals with high surface area and porosity. These materials exhibit unique physical and chemical properties that are beneficial in various applications, such as catalysis, sensors, and energy storage.2. Battery Materials: Nanoporous metals produced by electrochemical dealloying have been explored as anode materials for lithium-ion batteries. The high porosity and surface area of these materials enhance the lithium storage capacity and improve the battery's performance.3. Fuel Cells: Electrochemical dealloying has also been used to create nanostructured catalysts for fuel cells. These catalysts exhibit enhanced activity and durability, which are crucial for efficient fuel cell operation.4. Biomedical Applications: Nanoporous metals produced by electrochemical dealloying have potential applicationsin biomedicine, such as drug delivery, tissue engineering, and implant materials. The porous structure of these materials allows for controlled drug release and improved cell adhesion and growth.Challenges and Future Directions.Despite the significant progress made inelectrochemical dealloying, several challenges remain to be addressed. One of the primary challenges is the control of the dealloying process at the nanoscale, as it is crucialfor achieving the desired material properties. Additionally, the development of new electrolytes and optimization of dealloying parameters are ongoing research efforts.Future research in electrochemical dealloying could focus on exploring new alloy systems, optimizing the dealloying process for specific applications, and understanding the fundamental mechanisms underlying metal dissolution and nanostructure formation. Furthermore, the integration of electrochemical dealloying with other nanotechnology approaches, such as lithography and templating, could lead to the development of even more advanced materials with tailored properties.Conclusion.Electrochemical dealloying is a powerful technique for creating nanostructured materials with unique physical and chemical properties. Its applications span multiple fields, including materials science, energy conversion and storage, and biomedicine. While significant progress has been madein this field, there are still numerous challenges and opportunities for further research and development. With the advancement of nanotechnology and materials science, electrochemical dealloying holds promise for enabling thecreation of next-generation materials with improved performance and functionality.。
电化学分析讲义
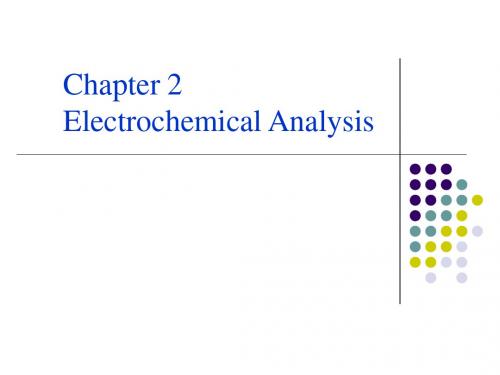
Potentiometric analysis Applying relationship between electrode
potential and activity or concentration of analyte to determine activity or concentration of analyte
②电解与库仑分析法 Electrolytic and coulometric analysis
Electrolytic analysis:
通过称量在电解过程中,沉积于电极表面的待测物 质量为基础的电分析方法。它是一种较古老的方法,又 称电重量法。此法有时可作为一种离子分离的手段。
One kind of electrochemical analysis based on weighing the product deposited on the surface of electrodes---also be referred to as electrogravimetry .
电池和电解池两类。 原电池(primary cell ,Galvanic or voltaic cell ):自
发的将电池内部进行的化学反应所产生的能量转化成电能 的化学电池。
电解池(electrolytic cell):实现电化学反应的能量由外 电源供给的化学电池,它是将电能转变为化学能。
溶液中的电流:正、负离子的移动。
The change of electrolyte conductivity can indicate the change of the concentration of the total ions; Direct conductometry and conductometric titration
- 1、下载文档前请自行甄别文档内容的完整性,平台不提供额外的编辑、内容补充、找答案等附加服务。
- 2、"仅部分预览"的文档,不可在线预览部分如存在完整性等问题,可反馈申请退款(可完整预览的文档不适用该条件!)。
- 3、如文档侵犯您的权益,请联系客服反馈,我们会尽快为您处理(人工客服工作时间:9:00-18:30)。
Journal of Hazardous Materials 148(2007)83–90Optimization of electrochemical treatment of industrial paintwastewater with response surface methodologyBahadır K.K¨o rbahti a ,Nahit Aktas ¸b ,Abdurrahman Tanyolac ¸c ,∗a Faculty of Engineering,Department of Chemical Engineering,University of Mersin,C ¸iftlikk¨o y 33343,Mersin,Turkey bEngineering &Architecture Faculty,Department of Chemical Engineering,Y¨u z¨u nc¨u Yıl University,65080Van,Turkeyc Faculty of Engineering,Department of Chemical Engineering,Hacettepe University,Beytepe 06800,Ankara,TurkeyReceived 28November 2006;received in revised form 2February 2007;accepted 5February 2007Available online 12February 2007AbstractThe electrochemical oxidation of water-based paint wastewater was investigated batch-wise in the presence of NaCl electrolyte with carbon electrodes for the first time in literature.The electrochemical treatment conditions were optimized using response surface methodology where potential difference,reaction temperature and electrolyte concentration were to be minimized while chemical oxygen demand (COD),color and turbidity removal percents and initial COD removal rate were maximized at 100%pollution load.The optimum conditions were satisfied at 35g/L external electrolyte concentration,30◦C reaction temperature and 8V potential difference (64.37mA/cm 2current density)realizing 51.8%COD and complete color and turbidity removals,and 3010.74mg/L h initial COD removal rate.According to these results,the electrochemical method could be a strong alterative to conventional physicochemical methods for the treatment of water-based paint wastewater.©2007Elsevier B.V .All rights reserved.Keywords:Electrochemical wastewater treatment;Paint wastewater;Graphite electrodes;Statistical experiment design;Response surface methodology;NaCl electrolyte1.IntroductionPaint is generally considered as a mixture of pigment,binder,solvent and additives.Paint classification can be made on many different bases;one convenient method is to classify paints based on their primary solvent for waste reduction and ing this approach,paints can be classified as water based,organic solvent based or powder (dry)and without solvent [1].Water-based paints have advantages over some types of organic solvent based coatings because they generally decrease VOC emis-sions,eliminate organic solvents for thinning and reduce the use of organic solvents during clean-up.The major waste that paint industry must manage is dominantly equipment-cleaning wastes,which makes up 80%of the waste generated in paint manufacture [2].∗Corresponding author.Tel.:+903122977404;fax:+903122992124.E-mail addresses:korbahti@.tr (B.K.K¨o rbahti),naktas@.tr (N.Aktas ¸),tanyolac@.tr (A.Tanyolac ¸).In recent years,electrochemical oxidation is becoming an alternative for wastewater treatment and starting to replace traditional processes,because many industrial processes pro-duce toxic wastewaters,which are not easily biodegradable and requiring costly physical or physico-chemical pretreatments [3].Many researchers had investigated the electrochemical oxida-tion of various types of wastewater containing 1,4-benzoquinone [3],phenol [4–6],olive oil [7],vinasse [8],p -chlorophenol and p -nitrophenol [9],nuclear wastes [10],human wastes [11],tannery wastewater [12]and textile wastewater [13,14].However,there is a lack of research dealing with electrochem-ical treatment of paint wastes in literature;present publications are only related to conventional treatment methods.A recent study by Kutluay et al.investigated the chemical treatability of water-based industry wastewater via adsorption and the authors concluded that highest COD removal efficiency was achieved with sodium bentonite [15].Another study by Dovletoglou et al.dealt with coagulation–flocculation of paint industry wastew-ater using ferrous and aluminum sulphate and polyaluminum chloride [16].El-Gohary et al.also applied chemical treatment0304-3894/$–see front matter ©2007Elsevier B.V .All rights reserved.doi:10.1016/j.jhazmat.2007.02.00584 B.K.K¨o rbahti et al./Journal of Hazardous Materials148(2007)83–90to paint factory wastewater for sedimentation using FeCl3in combination with CaO at optimum pH[17].The only work for biooxidation was carried out by Brown and Weintraub in a laboratory scale continuous-flow-through reactor using cathodic electrocoating paint process wastewater,which was not toxic at all to the microorganisms in activated sludge process[18].In electrochemical treatment,COD,color and turbidity removal in the presence of NaCl electrolyte were carried out mainly by indirect electrolysis generating in situ active chlorine (Cl2,HOCl/ClO−).The discharged chlorine gas(reaction(1)) [8,19–21]was in excess amount in the reactor and the irreversible reactions of hydrolysis(reaction(2))[19,21,22]and ionization take place(reaction(3))[19,22].Hypochlorous acid,HOCl,is a strong oxidant,which oxidizes the wastewater while OCl−is also consumed by reaction(4)[8,23,24]:2Cl−→Cl2+2e−(1) Cl2+H2O HOCl+H++Cl−(2) HOCl H++OCl−(3) 6OCl−+3H2O→32O2+6H++4Cl−+2ClO3−+6e−(4) In this study,electrochemical treatment of a simulated water-based paint wastewater was optimized in the presence of NaCl on carbon electrodes using response surface methodology by Design-Expert6(trial version).The runs were designed in accordance with the central composite design and carried out batch-wise.The water-based paint wastewater was synthetically prepared from industrial components for the standardization of the wastewater throughout the study.Five factors:pollution load percent,potential difference,electrolyte concentration,reaction temperature and reaction time were selected as effective process (independent)variables while COD,color and turbidity removal percents and initial COD removal rate as responses(dependent variable).2.Materials and methods2.1.Chemicals and materialsAcrylic copolymer water-based white primer was obtained from Marshall Boya Sanayi A.S¸.,Kocaeli,Turkey.Water-based blue colorant was supplied from Polisan Boya Sanayi ve Ticaret A.S¸.,Kocaeli,Turkey.Extra pure sodium chloride of Merck was utilized as additional electrolyte.Double distilled water was used for the preparation of synthetic water-based paint wastewater. All other chemicals used for the analysis were also obtained in highest degree of purity from various sources.2.2.Preparation and properties of synthetic water-based paint wastewaterThe100%polluted synthetic wastewater was prepared hav-ing46.15mL acrylic copolymer based white primer and4.62mL water-based blue colorant in a solution added up to1L with dou-ble distilled water,which corresponded to4.4%(w/v)mixture. The characteristics of simulated water-based paint wastewater is Table1The characteristics of simulated industrial water-based paint wastewater Parameter ValueChemical oxygen demand(mg/L)7496Turbidity(NTU)3378pH9.12Dissolved oxygen(mg/L) 6.16Total solids(mg/L)43,370Total suspended solids(mg/L)2770Total dissolved solids(mg/L)40,600Total volatile solids(mg/L)4460Non-volatile solids(mg/L)38,910Total solid content(%) 4.24Conductivity(S)394(=444.1S/cm) Density(g/cm3) 1.024presented in Table1whereas the original sample of discharged paint wastewater from a paint factory had values of COD,pH and total suspended solids as7863,7.84and3021mg/L,respec-tively,with4.4%(w/v)of total solid content.The characteristics of paint wastewater in literature studies were reported as,COD 459–19,000mg/L,pH6.7–7.5,turbidity500NTU,conductivity 1400S/cm,total suspended solids168–240mg/L[15–18,25]. In the runs for concentration effect,the wastewater was accord-ingly diluted in regard with the composition(100%)in Table1 with double distilled water.2.3.Experimental set-up and procedureThe electrochemical reactor system was used batch-wise in all runs.The reactor was made of Pyrex®glass having a net volume of600mL with a heating/cooling coil around.Three pairs of carbon electrodes(OD=12mm)were used as anode and cathode,and placed1.5cm apart on a Plexiglas®reactor cover.A glass stirrer with a single4cm×1.5cm rectangu-lar paddle was driven with a Heidolph-RZR1model mixer at575rpm.The reaction temperature was monitored with a glass thermometer immersed and controlled with circulating water recycled from a temperature controlled water bath(New Brunswick,Model G-86).The current was applied by a con-stant voltage/current controlled dc power source(NETES Model NPS-1810D).Synthetic paint wastewater was loaded into the reactor and the reaction under predetermined conditions started with the application of specified voltage and continuous agita-tion.At appropriate time intervals,samples of5mL were taken from the reactor and analyzed to determine the chemical oxygen demand,color,turbidity and pH.2.4.Experimental design and optimizationThe most popular class of second order designs called cen-tral composite design(CCD)was used for the response surface methodology in the experimental design.The CCD is ideal for sequential experimentation and allows a reasonable amount of information for testing lack offit while not involving an unusu-ally large number of design points[26].Therefore,the CCD withfive factors atfive levels was applied using Design-ExpertB.K.K¨o rbahti et al./Journal of Hazardous Materials148(2007)83–9085 Table2Experimental results of CCD designed experimentsRun no.x1(%)x2(V)x3(g/L)x4(◦C)x5(h)COD removal(%)Color removal(%)Turbidityremoval(%)COD initial removal rate(mg COD/h L)180812.540629.0098.9296.99681.84 280812.530625.2198.4997.081112.92 340837.540650.3697.9197.691934.82 480412.5306 5.5225.5118.13271.68 560625.035842.2897.9894.69546.92 640812.540226.5896.8695.94651.68 740837.540243.8098.5789.861934.82 840437.530223.1789.4086.91408.56 940437.530640.2997.7298.08408.56 1060625.035435.0999.1197.671576.12 1180837.530238.1797.8096.172761.76 1260625.035435.3999.1596.73546.92 1340412.5402 5.2340.5418.88250.44 146060.0354 6.7725.5335.40172.20 1560225.0354 1.2431.6010.57125.56 1660650.035449.3998.8896.05678.32 1740412.530210.3845.0333.31374.60 1860625.035436.0199.1197.63836.64 1960625.035432.8699.3797.92948.44 2060625.035425.9798.0196.85167.28 2140437.540623.7594.9195.39414.24 2240437.540210.4678.4467.86414.24 23100625.035429.0999.7599.08667.92 24601025.035446.3598.9096.782600.80 2580437.540618.5493.8184.84368.96 2680837.530654.7799.0099.772761.76 2760625.035435.0999.1197.671576.12 2880412.5302 4.1318.1116.88271.68 2920625.035449.3298.1394.86791.84 3040812.540626.9896.8697.45651.68 3140837.530248.9596.5796.101477.84 3240837.530665.6898.7496.561477.84 3360625.045427.1198.9896.63661.08 3480412.5402 3.0237.4712.57244.48 3580437.5402 5.6556.4134.67368.96 3660625.035436.0199.1197.63836.64 3760625.025436.0998.1294.33938.64 3860625.03500000 3940412.540613.0770.1961.33250.44 4080837.540242.2498.9495.783160.96 4140812.530642.4998.4397.361028.12 4260625.035432.8699.3797.92948.44 4380812.530215.1780.5079.741112.92 4480837.540652.7999.4698.053160.96 4580437.530624.3596.5094.36675.20 4680412.540611.4980.5157.71244.48 4740812.530231.6491.5589.531028.12 4880437.53028.0546.7324.86675.20 4940412.530614.0065.5251.53374.60 5080812.540217.8784.5375.63681.846.0.Each independent variable was coded atfive levels between −2and+2,where the variables pollution load(x1),potential dif-ference(x2),electrolyte concentration(x3),reaction temperature (x4)and reaction time(x5)were changed in the ranges20–100%, 2–10V,0–50g/L,25–45◦C and0–8h,respectively.The critical ranges of selected parameters were determined by preliminary experiments based on literature experience.Forty-two experiments were augmented with eight replica-tions at the design center to evaluate the pure error and were carried in randomized order as required in many design pro-cedures.First six columns of Table2show run number and experimental conditions of the runs arranged by the CCD. Performance of the process was evaluated by analyzing the responses;COD,color and turbidity removal percents and COD initial removal rate.In the optimization process,the responses are related to fac-tors by quadratic models,which also include the linear model as shown in Eq.(5),whereηis the response,x i and x j are vari-86 B.K.K¨o rbahti et al./Journal of Hazardous Materials 148(2007)83–90ables,β0the constant coefficient,βj s,βjj s and βij s are interactioncoefficients of linear,quadratic and the second-order terms,respectively,and e i is the error:η=β0+k j =1βj x j +k j =1βjj x 2j +i k <j =2βij x i x j +e i(5)COD,color,turbidity removal percent and initial COD removalrate data were processed for Eq.(5)using Design-Expert 6.0pro-gram including ANOV A to obtain the interaction between the process variables and the response.The coefficients of determi-nation R 2and R 2adj expressed the quality of fit of the resultant polynomial model,and statistical significance was checked by F -test in the program.For optimization,a module in Design-Expert software searched for a combination of factor levels that simultaneously satisfy the requirements placed on each of the responses and factors.The desired goals were selected as maxi-mum COD,turbidity and color removal percents,and maximum initial COD removal rate at minimum reaction temperature,elec-trolyte concentration and potential difference within the range of reaction time at 100%pollution load.Corresponding impor-tances of goals were selected as 5for COD,color and turbidity removal percents and initial COD removal rate;3for pollution load,potential difference,electrolyte concentration and reac-tion time,and 1for reaction temperature.These individual goals were combined into an overall desirability function by Design-Expert software for maximization to find the best local maximum [27].2.5.AnalysisDuring the reaction 5mL samples were taken from the elec-trochemical reactor at appropriate time intervals and pH was measured with a NEL pH30model pH meter,then centrifuged at 5000rpm for 10min to have supernatant for analysis and mea-surements.The color of the reaction medium was monitored by a Hitachi 150-20model spectrophotometer at 595nm and the tur-bidity was measured by a Hach 2100AN IS model turbidimeter at 860nm.COD analysis performed with Palintest PL464test kit after color and turbidity analysis.In order to prevent the pre-cipitation of Ag +ions in COD test kits with Cl −ions present in the sample,the samples were pre-treated with appropriate volumes of 2M AgNO 3prior to COD analysis.AgCl precipi-tated and the sample was centrifuged at 5000rpm for 10min.The supernatant was removed and the precipitate was washed with 0.5mL pure sulphuric acid for desorption of the adsorbed waste and centrifuged at 5000rpm for 10min.This procedure was repeated twice.All of the collected supernatants were volu-metrically measured,mixed and 1mL of the sample mixture was added to COD kit for digestion.Grant QBT2model block heater was set to 150◦C and sample and blank tubes were digested for 2h.Then the tubes were allowed to cool down to room tem-perature and the absorbance of the samples was read at 605nm.The COD of the samples was calculated using a calibration curve prepared previously taking the dilutions into account in washingsteps.Fig.1.COD,color and turbidity removal profiles for run 32(pollution load:40%;potential difference:8V;electrolyte concentration:37.5g/L;reaction tem-perature:30◦C).3.Results and discussionFifty CCD designed batch runs were conducted to visual-ize the effects of independent factors on responses and the results along with the experimental conditions were presented in Table 2.More than 35%COD removal was realized in 20runs while over 90%color and turbidity removals were achieved in more than 30runs of Table 2.As a general trend,increase in electrolyte concentration and potential difference resulted in enhanced COD,color and turbidity removals and COD initial removal rate in all runs.In Table 2,run 32specifically gave the best removal for COD as 65.68%while more than 95%removal of color and turbidity was achieved in the same run after 8h,although initial COD removal rate was not realized as high as in runs 40and 44per-formed at 40◦C.Fig.1shows COD,color and turbidity removal percent and pH time profiles in this run.In Fig.1,above 90%of color and turbidity removal achieved within 2h of reaction time indicating more efficient removal of color and turbidity than that of COD.In Fig.1,medium pH decreased from 10.2to 7.0within 3h,being an advantage for neutralization of treated water without using any chemical.This trend was observed in all runs and was most likely due to hydrolysis,ionization and OCl −consumption reactions,in which H +is a by-product [21].3.1.Evaluation of experimental results with Design-Expert Experimental results were evaluated with Design-Expert 6.0using approximating functions of dependent variables COD (y 1),color (y 2),turbidity (y 3)removal percents and initial COD removal rate (y 4).These approximating functions are presented in Eqs.(6)–(9):y 1=−23.770−1.436x 1+10.705x 2+0.994x 3+0.974x 4+9.960x 5+0.0027x 21−0.692x 22−0.0109x 23−0.0327x 24−0.858x 25−0.0013x 1x 2−0.0004x 1x 3+0.0255x 1x 4+0.0086x 1x 5+0.118x 2x 3+0.0194x 2x 4+0.0152x 2x 5−0.0203x 3x 4+0.0704x 3x 5−0.0672x 4x 5(6)B.K.K¨o rbahti et al./Journal of Hazardous Materials148(2007)83–9087y2=−22.689−2.756x1+39.895x2+6.360x3−5.727x4 +27.718x5+0.0057x21−1.533x22−0.0441x23+0.0878x24−2.549x25+0.0850x1x2+0.0022x1x3+0.0300x1x4+0.0690x1x5−0.288x2x3−0.177x2x4−1.328x2x5−0.0434x3x4−0.0308x3x5+0.0831x4x5(7) y3=−86.406−2.232x1+47.709x2+6.106x3−3.994x4 +25.306x5+0.0038x21−2.328x22−0.0403x23+0.0456x24−2.723x25+0.116x1x2−0.0012x1x3+0.0169x1x4+0.0731x1x5−0.345x2x3−0.0441x2x4−1.588x2x5−0.0308x3x4+0.0218x3x5+0.215x4x5(8)y4=6445.725−30.048x1−865.595x2−121.539x3−129.600x4+174.846x5+0.0263x21+42.210x22−0.420x23+1.120x24−20.669x25+3.926x1x2+0.681x1x3−0.205x1x4+12.838x2x3+3.129x2x4+1.514x3x4(9) In Eqs.(6)–(9)x1,x2,x3,x4,and x5are corresponding to independent variables of pollution load,potential difference, electrolyte concentration,reaction temperature and reaction time,respectively.ANOV A results of these quadratic models are presented in Table3.In the table,model F-values of34.93,9.92, 15.50and10.25imply the models are significant for COD,color, turbidity removal percents and initial COD removal rate,respec-tively.For all equations,adequate precision signal-to-noise ratio is greater than4,which is desirable for sound models.Also for all models,Prob>F is less than0.0001,indicating that terms are significant in all models while correlation coefficient changed in the range0.94–0.98denoting good enough quadraticfits to nav-igate the design space.Normal%probability and studentized residuals graphs for responses y1–y4yielded fair straight lines, proving normal distribution of the data.3.2.Optimization of experimental conditionsThe results were optimized by Design-Expert software using the approximating functions in Eqs.(6)–(9).In optimization,a cost driven approach was preferred in the range of25–45◦C reaction temperature within0–8h of reaction time;potential difference,reaction temperature and electrolyte concentration were to be minimized so as to save energy and electrolyte, whereas COD,color and turbidity removal percents and ini-tial COD removal rate were maximized at100%pollution load. The optimization results are shown in Table4in accord with descending desirability whereas PD,EC,T,t,CR,ICR and D are corresponding to potential difference,electrolyte concentra-tion,reaction temperature,reaction time,COD removal percent, initial COD removal rate and desirability,respectively.Optimized conditions under specified constraints were obtained for highest desirability at100%pollution load,35g/L electrolyte concentration,30◦C reaction temperature and8V potential difference(64.37mA/cm2current density)after6.79h of reaction time.Under these optimized conditions,51.8%COD removal,100%color and turbidity removals and3010.74mg/L h initial COD removal rate were estimated.In order to validate the optimization,a specific batch run was performed under these optimum conditions.In this run,COD,color and turbidity removals,and initial COD removal rate were realized as68.7%, 99.3%,99.1%and2748.9mg/L h,respectively,proving predic-tive power of the model approach,although COD removal was underestimated.3.3.Effects of operational parameters at optimum conditionsEqs.(6)–(9)have been used to visualize the effects of experimental factors on responses under optimized conditions in3D graphs of Figs.2–6.In Fig.2,COD removal percent increased by increasing electrolyte concentration and poten-tial difference above11.8g/L and4V(5.16mA/cm2current density),respectively,and more than60%COD removal was realized at100%pollution load and30◦C reaction tempera-ture within8h of reaction time above7.5V potential difference (43.88mA/cm2current density)and38.9g/L electrolyte con-centration.Increasing electrolyte concentration and voltage increased the conductivity and current simultaneously,which in turn enhanced the reaction rate of electrochemical destruction. Moreover,since the presence of NaCl in the reaction medium generates in situ very strong oxidants of HOCl/ClO−increase of electrolyte concentration and voltage directly increase the concentration of these chemicals in the medium enabling faster COD,color and turbidity removal[8,19,21–24].In Fig.3,COD removal decreased below80%pollution load by increasing reaction temperature but was not affected above this percent.At low temperatures COD removal increasedby Fig.2.The effect of potential difference and electrolyte concentration on COD removal(pollution load:100%;reaction temperature:30◦C;reaction time:8h).88 B.K.K¨o rbahti et al./Journal of Hazardous Materials148(2007)83–90Table3ANOV A results of the quadratic models for COD,color and turbidity removal percents and initial COD removal rateSource Sum of squares Degrees of freedom Mean square F-Value Prob>F COD removal(%)Model12742.6320637.1334.93<0.0001 Residual528.972918.24Lack offit450.422220.47 1.820.2111 Pure error78.54711.22R2=0.96,R2adj=0.93,adequate precision=25.59Color removal(%)Model31175.46201558.779.92<0.0001 Residual4554.7529157.06Lack offit4553.4422206.971105.68<0.0001 Pure error 1.3170.19R2=0.87,R2adj=0.78,adequate precision=13.01Turbidity removal(%)Model42795.99202139.8015.50<0.0001 Residual4004.7129138.09Lack offit4003.2522181.97872.59<0.0001 Pure error 1.4670.21R2=0.91,R2adj=0.86,adequate precision=14.79Initial COD removal rate(mg/L h)Model 2.749×10720 1.374×10610.25<0.0001 Residual 3.888×10629 1.341×105Lack offit 2.307×10622 1.048×1050.460.9201 Pure error 1.582×1067 2.259×105R2=0.88,R2adj=0.79,adequate precision=12.17pollution load implying a positive order of magnitude of waste concentration in overall kinetics.As a general rule,the reaction rate increases as the temperature increases,however the increase in temperature did not have a significant effect on COD removal, in fact caused a visible drop for lower COD load percents.In Fig.3,higher COD removal was realized at lower reaction tem-peratures.At higher temperatures with low pollution load,this behavior could be attributed to the inhibition of electrochemical oxidation and/or increasing corrosion rate of the electrodes as well as to more conversion of electrical energy into heat due to increased resistance of the medium at high temperatures. Above90%pollution load at30◦C,8V potential difference and35g/L electrolyte concentration,a region of more than50% COD removal was achieved within8h of reaction time.In lit-erature there is limited number of studies for comparison,but they were accomplished via biodegradation and mostly coagula-tion/sedimentation,not by electrochemical destruction.Brown and Weintraub obtained COD reduction as39%and87%for residence times of1.2and2.4days,respectively,with activated sludge treatment of paint process wastewater[18].Huang and Ghadirian applied coagulation/flocculation to wastewater sam-ples from a paint manufacturing plant,and they obtained70% COD removal at pH3.5,while only35%removal was achieved at alkaline pH[25].Dovletoglou et al.determined the optimum pH9.7for FeSO4addition at a dose of2g/L,and achieved 30–80%COD removal via coagulation/flocculation while no pH adjustment was needed using Al2(SO4)3at a dose of2.5g/L, which yielded70–95%COD removal[16].El-Gohary et al.also applied physicochemical treatment to the paint factory wastew-ater using50mg/L FeCl3in combination with140mg/L CaO at an optimum pH8.2and they obtained90%COD removal[17]. Kutluay et al.investigated treatability of water-based industrialTable4Optimization results for maximum treatmentSolution no.PD(V)EC(g/L)T(◦C)t(h)CR(%)Color removal(%)Turbidity removal(%)ICR(mg/L h)D 17.9636.4429.8 6.7951.81001003007.880.64 27.9636.4329.8 6.8151.71001003006.160.64 37.9636.4129.8 6.7851.71001003002.940.64 47.9636.4429.8 6.7851.81001003005.610.64 57.9636.4529.9 6.8051.81001003010.740.64 67.9636.4029.9 6.8051.81001003005.310.64 77.9536.4629.9 6.7951.81001003004.110.64B.K.K¨o rbahti et al./Journal of Hazardous Materials 148(2007)83–9089Fig.3.The effect of reaction temperature and pollution load on COD removal(potential difference:8V;electrolyte concentration:35g/L;reaction time:8h).paint wastewater by adsorption using sodium bentonite.Their work resulted in 84–94%COD removal with 500mg/L sodium bentonite concentration at original pH of the wastewater [15].In terms of COD removal,some of literature studies apparently achieved better results than this work,however,this success was granted at the expense of rather high concentration of coagulants and adsorbents requiring pH adjustment and their processes were merely separation rather than destruction.In Fig.4,above 7V potential difference (32.33mA/cm 2cur-rent density)complete color removal was obtained between 9.4and 44.9g/L electrolyte concentration at 100%pollution load and 30◦C reaction temperature within 3h.Kutluay et al.achieved 92–100%color removal using sodium bentonite with 300–1000mg/L dosages at pH 9,10and original wastewater pH.In Fig.5,complete turbidity removal was obtained above 7.4V potential difference (41.28mA/cm 2current density)for 5.5–40.2g/L electrolyte concentration at 100%pollutionloadFig.4.The effect of potential difference and electrolyte concentration on color removal (pollution load:100%;reaction temperature:30◦C;reaction time:3h).Fig.5.The effect of potential difference and electrolyte concentration on turbid-ity removal (pollution load:100%;reaction temperature:30◦C;reaction time:3h).Fig.6.The effect of reaction temperature and pollution load on turbidity removal (potential difference:8V;electrolyte concentration:35g/L;reaction time:3h).and 30◦C reaction temperature within 3h of reaction time.In Fig.6within 3h reaction time,complete turbidity removal was realized below 33◦C at 100%pollution load,8V potential difference,35g/L electrolyte concentration.In literature,Dovle-toglou et al.achieved turbidity removal as 70–99%,90–99%and 98%with the addition of FeSO 4,Al 2(SO 4)3and polyaluminum chloride,respectively,with the addition of coagulant for 4min,flocculation for 25min and settling for 30min of time,which is shorter than our removal rate [16].4.ConclusionThe electrochemical treatment of synthetic industrial water-based paint wastewater was studied in a batch reactor with carbon electrodes in the presence of NaCl electrolyte.The high-est removals for COD,color and turbidity were realized 65.68%。