Plant Cell-2012-Gao-1437-47[1]
植物细胞工程- PPT课件

植物细胞工程 热点
• 1.在无菌和人工控制的条件下,采用植物细胞 和组织培养技术培养植物的细胞,组织,器 官以获得有药用价值的次生代谢产物。 2.采用植物的遗传操作技术改变植物或植物细 胞的原有性状和功能,获得具有优良性状的 植株或植物细胞,生产有药用价值的次生代 谢产物。
第二节 植物细胞培养特性 及主要培养技术
三、植物细胞的培养特点
(1)植物细胞有独特的培养时间及特征:重量的 增加在对数期,次生代谢产物积累在稳定期 (2)植物细胞很少单一细胞生长,对数生长后期分 泌量蛋白和粘多糖,以非均相集合的细胞团悬浮 生长。
(3)植物细胞好气,培养过程需供气及搅拌,
(4)植物细胞较微生物细胞大得多,纤维素细胞壁, 细胞壁脆弱,抗剪切能力小,传统的搅拌式反应 器极易损伤细胞壁
(2)无机氮源,硝酸盐,铵盐为主。
(3)碳源,蔗糖 (4)需多种维生素烟酸,B1,B6和植物生长激素 生
长素,细胞分裂素,赤霉素,脱落酸,乙烯。
(5)需有机物:甘氨酸或水解络酪蛋白,酵母提取液
等。
A
B
A:BA(0mg/L) ,芽(减少),根(增多)愈伤组织(一定量) B:BA(0.1mg/L),芽(适量),根(适量)愈伤组织(一定量)
二、培养植物细胞 四个生长时期
延迟期:细胞数恒定,高RNA含量,高蛋白质合 成能力; 加速期:细胞快速生长期。细胞数、DNA和蛋白 质浓度增加,有丝分裂活性、RNA含量和蛋白质 合成能力减少; 对数期:介于最大生长率和蛋白质合成完全停止 期之间。增加细胞鲜重、干重及RNA酶活性,蛋 白质合成能力完全减退; 稳定期:细胞数稳定。细胞高液泡化,极度脆弱, 高度分化及高浓度有机化合物。
第四章 植物细胞工程 Plant Cell Engineering
药用植物学 第1章 Plant Cells

Fiber cell of flax(亚麻)
4cm long
Fiber of ramee(苎麻)
55cm long
2.Basic Structure and Function of Plant cells Definition
Microscopic structure: there can be seen the cell
pound starch grain
Plasmodesmata of Persimmon core
Ⅲ. Specification of Cell Walls
lignification suberization cutinization mucilagization mineralization
1.lignification: The cell wall has increased more lignin (木质素)while adding and growing. ①Distinguish: it was stained Red when adding meta benzene and three phenol + dense hydrochloric acid is. ②Example: vessel(导管) , wood fiber , stone cell.
Ⅰ.starch grain: hilum , annular striation
lamellae reserve starch: ①simple starch grain ②compound starch grain ③half compound starch grain
1.2.3.4.simple starch grain (mbdoidal(人字形的) hilum 2.short gap(短缝状) )
植物学之植物组织系统

外膜
内膜
基粒
基质片层
是单层膜围成的扁平小囊,膜上含有光合色素,又称光合膜。
基质
Structure and function of chroloplast
外膜的渗透性大,物质可自由进出。
内膜对通过物质的选择性很强,控制物质的进出
类囊体
许多类囊体象圆盘一样叠在一起,称为基粒。
贯穿在两个或两个以上基粒之间的没有发生垛叠称为基质片层或基质类囊体。
Form and size of plant cell
basic structure of plant cell
植物细胞的基本结构
原生质体
细胞壁
后含物
细胞膜 细胞质 细胞核
植物细胞
基质 双层膜细胞器 单层膜细胞器 内膜系统 细胞骨架 核糖体
显微结构 超微结构
60年代末,扫描电子显微镜(scanning electron microscopy,SEM)问世并被广泛应用,使人们能直接观察到生物,乃至细胞立体、生物的结构。 ★
60年代,组织培养技术→细胞全能性: 证明细胞学说
1
2
3
4
5
morphology and structure of plant cell 2.1 plant cell form 植物细胞的形状
线粒体
Function:
endoplasmic reticulum
粗糙型内质网(rough ER):参与蛋白质的合成与运输功能
光滑型内质网(smooth ER):主要合成和运输类脂和多糖 分布于细胞质中的网状膜系统,由管状、囊泡状或片状结构的膜构成。
Golgi body 高尔基体 (高尔基器)
由一叠扁平的囊(cisterna)组成的结构,直径约0.5—1μm,囊的边缘产生囊状管,相互交织成网状。周围由管通过缢缩断裂,形成高尔基小泡(vesicle),小泡可从高尔基体囊上分离出去。
Plant Cell-2011

BENT UPPERMOST INTERNODE1Encodes the Class II Formin FH5Crucial for Actin Organization and Rice DevelopmentW OAWeibing Yang,a,1Sulin Ren,b,1Xiaoming Zhang,c,1Mingjun Gao,a Shenghai Ye,c Yongbin Qi,c Yiyan Zheng,b Juan Wang,b Longjun Zeng,a Qun Li,a Shanjin Huang,b,2and Zuhua He a,2,3a NationalKey Laboratory of Plant Molecular Genetics,Institute of Plant Physiology and Ecology,Chinese Academy of Sciences,Shanghai 200032,Chinab Key Laboratory of Photosynthesis and Environmental Molecular Physiology,Institute of Botany,Chinese Academy of Sciences,Beijing 100093,Chinac State Key Laboratory Breeding Base for Zhejiang Sustainable Pest and Disease Control,Zhejiang Academy of Agricultural Sciences,Hangzhou 310021,ChinaThe actin cytoskeleton is an important regulator of cell expansion and morphogenesis in plants.However,the molecular mechanisms linking the actin cytoskeleton to these processes remain largely unknown.Here,we report the functional analysis of rice (Oryza sativa )FH5/BENT UPPERMOST INTERNODE1(BUI1),which encodes a formin-type actin nucleation factor and affects cell expansion and plant morphogenesis in rice.The bui1mutant displayed pleiotropic phenotypes,including bent uppermost internode,dwarfism,wavy panicle rachis,and enhanced gravitropic response.Cytological observation indicated that the growth defects of bui1were caused mainly by inhibition of cell expansion.Map-based cloning revealed that BUI1encodes the class II formin FH5.FH5contains a phosphatase tensin-like domain at its amino terminus and two highly conserved formin-homology domains,FH1and FH2.In vitro biochemical analyses indicated that FH5is capable of nucleating actin assembly from free or profilin-bound monomeric actin.FH5also interacts with the barbed end of actin filaments and prevents the addition and loss of actin subunits from the same end.Interestingly,the FH2domain of FH5could bundle actin filaments directly and stabilize actin filaments in vitro.Consistent with these in vitro biochemical activities of FH5/BUI1,the amount of filamentous actin decreased,and the longitudinal actin cables almost disappeared in bui1cells.The FH2or FH1FH2domains of FH5could also bind to and bundle microtubules in vitro.Thus,our study identified a rice formin protein that regulates de novo actin nucleation and spatial organization of the actin filaments,which are important for proper cell expansion and rice morphogenesis.INTRODUCTIONRice (Oryza sativa )is a major food resource for nearly half of the world human population.Rice productivity is highly associated with its architectural pattern,including plant height,which is attributable mainly to stem internode elongation (Sasaki et al.,2002;Wang and Li,2008).The uppermost internode is of particular importance for rice productivity,since the elongation of the uppermost internode promotes panicle emergence (Zhu et al.,2006).The phytohormones gibberellins (GAs)and brassinosteroids are the two major factors that affect rice internode length by modulating cell expansion (Wang and Li,2008).The cytoskeleton,including microtubules and actinmicrofilaments,is also essential for plant development and morphogenesis by modulation of cell expansion.For example,loss of function of DWARF AND GLADIUS LEAF1,which encodes an ATPase katanin-like protein in rice,caused disor-ganization of microtubule arrays and inhibited cell elongation,resulting in a dwarf phenotype (Komorisono et al.,2005).However,the information about the functions of the actin cyto-skeleton in cell elongation and rice morphogenesis is rather limited.Pharmacological perturbation of actin organization indicates that the actin cytoskeleton is a major regulator of cell elongation in Arabidopsis thaliana and other plant species (Baluska et al.,2001;Collings et al.,2006).Simultaneous downregulation of ACTIN2and ACTIN7reduced cell elongation in Arabidopsis hypocotyls (Kandasamy et al.,2009).Misexpression of actin regulatory proteins,such as profilin and actin-depolymerizing factors,also perturbs cell elongation (Ramachandran et al.,2000;Dong et al.,2001;Kandasamy et al.,2009).In addition,the actin cytoskeleton plays pivotal roles in polar cell expansion and the establishment of cell division planes by governing cytoplasmic streaming,organelle movement,and vesicle trans-port (Martin et al.,2001;Staiger and Blanchoin,2006).However,1These authors contributed equally to this work.2These authors contributed equally to this work.3Address correspondence to zhhe@.The authors responsible for distribution of materials integral to the findings presented in this article in accordance with the policy described in the Instructions for Authors ()are:Shanjin Huang (sjhuang@)and Zuhua He (zhhe@).WOnline version contains Web-only data.OAOpen Access articles can be viewed online without a /cgi/doi/10.1105/tpc.110.081802This article is a Plant Cell Advance Online Publication.The date of its first appearance online is the official date of publication.The article has been edited and the authors have corrected proofs,but minor changes could be made before the final version is published.Posting this version online reduces the time to publication by several weeks.The Plant Cell Preview, ã2011American Society of Plant Biologists1of 20the molecular mechanisms by which actin regulates these phys-iological processes remain poorly understood.The function of the actin cytoskeleton is tightly coupled with its dynamic properties(Traas et al.,1987).Actin dynamics include maintenance of the monomeric actin(G-actin)pool,nucleation, actinfilament assembly and disassembly,actin bundle forma-tion,and actin cable construction,which are modulated by a precise orchestration of the activities and functions of a plethora of actin binding proteins(Staiger and Blanchoin,2006;Higaki et al.,2007).Nucleation is the rate-limiting step during sponta-neous actinfilament assembly(Pollard and Borisy,2003).To date,several actin nucleation factors have been identified, including the Actin-Related Protein2/3complex,formins,Spire, Cordon-bleu,Leiomodin,and Junction-Mediating and Regula-tory protein,which allow the cell to determine when and where to polymerize actinfilaments(Baum and Kunda,2005;Quinlan et al.,2005;Ahuja et al.,2007;Chereau et al.,2008;Zuchero et al.,2009).Formins,originally identified from a mouse limb deformity mutant,have been found to exist in many eukaryotic organisms, including animals,fungi,and plants,and are involved in many fundamental cellular processes,including cytokinesis,cell mo-tility,and polarity(Woychik et al.,1990;Goode and Eck,2007). Formins are multidomain-containing proteins,characterized by two highly conserved formin-homology domains,FH1and FH2. Some formins in fungi and animals also share additional con-served domains such as the FH3domain,the Rho binding domain,the Diaphanous-autoregulatory domain,and the Diaph-anous-inhibitory domain,which confer functional regulation of these formins(Goode and Eck,2007).The FH1domain,con-sisting of several consecutive polyproline stretches,binds pro-filin or profilin/actin complexes to induce actin polymerization from the barbed end(Pruyne et al.,2002;Kovar et al.,2006).The number of polyproline stretches differs among formin proteins. The FH2domain contains actin binding sites and acts as a dimer to nucleate new actinfilaments(Pruyne et al.,2002;Xu et al., 2004;Otomo et al.,2005).General activities of formins include nucleating actin assembly and interacting with the barbed end of actinfilaments(Kovar,2006;Goode and Eck,2007).Some formins also have severing and bundling activities(Harris et al., 2004;Michelot et al.,2005;Moseley and Goode,2005;Harris et al.,2006).Along with their functions in regulating actin cyto-skeleton organization,several animal formins,including mDia1, mDia2,Cappuccino(Capu),and Inverted Formin1(INF1),have been shown to bind directly to microtubules,thus regulating their dynamic properties(Palazzo et al.,2001;Rosales-Nieves et al., 2006;Bartolini et al.,2008;Young et al.,2008;Miki et al.,2009). The plant formin At FH4also associates with microtubules via the unique group Ie domain,which is located between the trans-membrane domain and the FH1domain(Deeks et al.,2010), suggesting that these formins may function in the crosstalk between microtubule and actin cytoskeleton systems.Plant genomes encode a large family of formins that do not share recognizable autoregulatory domains found in animal and yeast formins(Grunt et al.,2008).Based on sequence similarity and conservation,plant formins are divided into two classes, referred to as class I and class II.Class I formins have an N-terminal membrane-anchoring domain followed by a transmembrane region and C-terminal FH1and FH2domains,whereas class II formins carry an N-terminal phosphatase and tensin-related (PTEN)-like domain besides the conserved FH1and FH2do-mains(Deeks et al.,2002;Cvrckova´et al.,2004;Grunt et al., 2008).Functional analysis of class I formins has shown that they are involved in many fundamental physiological processes.For instance,a loss-of-function mutation of At FH5leads to defective cytokinesis in seed endosperm(Ingouff et al.,2005).At FH5was recently shown to stimulate actin assembly from the subapical domain of pollen tubes to facilitate pollen tube growth,and downregulation of FH5inhibits tip growth of pollen tubes in Nicotiana tabacum(Cheung et al.,2010).Downregulation of AFH3causes depolarized pollen tube growth,whereas over-expression of AFH1arrests pollen tube tip growth,and over-expression of At FH8disturbs root hair tip growth(Cheung and Wu,2004;Yi et al.,2005;Ye et al.,2009).By contrast,functional studies of class II formins are relatively limited.Recent results from the moss Physcomitrella patens demonstrated that class II formins could elongate actinfilaments at an amazingly rapid rate and are essential for polar cell expansion(Vidali et al.,2009). Most recently,a class II formin,AFH14,was reported to bind both microtubules and microfilaments and was shown to play important roles in cell division and microspore formation in Arabidopsis(Li et al.,2010).However,it remains a major chal-lenge to explore the physiological functions of each formin protein in plants,particularly in the model cereal rice,for which no single formin has been functionally characterized.We previously reported the identification of the rice ELON-GATED UPPERMOST INTERNODE(EUI)gene encoding a P450 that deactivates bioactive GAs.Mutation of Eui led to increased bioactive GA levels and an extremely elongated uppermost internode(Zhu et al.,2006).To understand rice internode devel-opment better,we characterized a rice mutant with a bent uppermost internode,designated as bent uppermost internode1 (bui1).The bui1mutant displayed multiple defects in plant architecture,including a bent uppermost internode,short culms, high tillering,wavy panicle rachises,and enhanced gravitropism. Map-based cloning revealed that BUI1encodes the class II formin FH5.Cytological and biochemical analyses demonstrated that FH5/BUI1plays essential roles in diffuse cell expansion and rice morphogenesis.RESULTSPhenotypic Characterization of the bui1MutantTo understand better the cellular mechanism underlying rice internode development,we isolated a nonclassic dwarfed mu-tant from a g-ray–induced mutation pool(Zhu et al.,2006).The mutant was named bui1based on its bent uppermost internode phenotype(see below).Compared with wild-type plants,bui1 had more tillers but significantly reduced plant height(Figure1A; see Supplemental Figures1A and1B online).During the early heading stage,the bui1uppermost internodes were much shorter than those of the wild-type plants,and young panicles of bui1could hardly break out of theflag leaf sheath(Figure1B). Longitudinal section analysis revealed that the cells of the bui12of20The Plant Celluppermost internode were markedly shorter and swelled com-pared with the wild-type cells (Figures 1C and 1D;see Supple-mental Figure 1C online).These results indicate that cell elongation was inhibited by the bui1mutation.Although the bui1panicles could eventually protrude from the leaf sheath,they were small and loose,exhibiting a cockscomb shape (Figure 1B).The uppermost internode of bui1did not grow upward;instead,it was bent almost perpendicularly to the vertical axis (Figure 1E).To understand fully the cellular basis underlying the bending phenotype,we analyzed cell morphology in the middle part of the uppermost internode,where shoot bending was most severe.Longitudinal section analysis revealed that,in addition to the shorter and slightly swollen cell shapes,bui1also displayed other cellular phenotypes.In the wild-type plants,cell margins were even and connected tightly with each other (Figure 1F),whereas cell boundaries in bui1were rough,with obvious protuberances along the margins and ruptures in cell-to-cell boundaries (Figure 1G).Compared with the rectan-gular cells in the wild type,most bui1cells were slanted,and cellfiles were not aligned properly (Figure 1H).Taken together,these results indicate that polar cell expansion was also disrupted in bui1.The bui1mutant displayed other morphological defects be-sides the bent uppermost internode.All primary and secondary rachises of bui1were wavy,and the number of rachis branches and spikelets were reduced compared with the wild-type plants (Figure 1I).The seeds of bui1were smaller with irregular shapes and were not well filled (Figure 1J).Mutation of BUI1Affects Seedling Development and GravitropismWild-type young seedlings display erect upward growth due to negative gravitropism,whereas bui1seedlings bend in all direc-tions (Figure 2A),suggesting an altered gravitropic response in bui1.The bending of bui1under normal growth conditions makes it difficult to analyze shoot gravitropism.We thus analyzed the gravitropic response of the roots instead.The kineticsofFigure 1.Phenotypes of Wild-Type and bui1Mature Plants.(A)Wild-type (Zhejing 22;left)and bui1(right)plants.Bar =2cm.(B)Panicle exsertion of wild-type (left three)and bui1(right three)plants.Bar =2cm.(C)and (D)Longitudinal sections of the uppermost internodes of the wild type (C)and bui1(D)at heading stage.The regions used for analysis are indicated with squares in (B).Bars =100m m.(E)Panicles of the wild type (left)and bui1(right)at the mature stage.Bar =2cm.(F)to (H)Longitudinal sections of the uppermost internode regions (indicated with squares in [E])of the wild type (F)and bui1(G).Severely slanted cells of the same bui1internode region are shown in (H).Bars =50m m.(I)Panicle rachis of the wild type (left)and bui1(right).Note the wavy rachis in bui1.Bar =2cm.(J)Grains of the wild type (top)and bui1(bottom).Bar =0.5cm.Rice FH5Regulates Actin Organization 3of 20gravity-induced root bending of light-grown seedlings revealed that gravitropism in bui1was strongly promoted,with an accel-erated response to the gravitropic stimulus and an increased bending degree (see Supplemental Figure 2online).The shoots of bui1seedlings were shorter than those of wild-type plants (Figure 2B;see Supplemental Figure 3A online).Longitudinal section analysis revealed that cell elongation was inhibited in the bui1shoot (Figures 2C and 2D;see Supplemental Figure 3B online).Interestingly,when cultured in half-strength Murashige and Skoog medium,bui1roots displayed a strong wavy phenotype,with root length only slightly reduced (Figure 2E;see Supplemental Figure 3C online).To gain insight into the cellular mechanism for the wavy-root phenotype,we analyzed the internal structure of bui1and wild-type roots.These analyses revealed that cells in the bui1roots were slanted with reduced cell length but little change in cell width (Figures 2F and 2G;see Supplemental Figure 3D online).Cell files corresponding to the wavy region also exhibited a wavy manner,but they stillremained parallel to each other (Figure 2G),suggesting that this wavy growth behavior is different from the twisted growth in the Arabidopsis microtubule-related mutants (Ishida et al.,2007).We speculated that bui1might be defective in normal root thigmot-ropism,as observed in the Arabidopsis mildew resistance locus o4mutant (Chen et al.,2009).Based on these observations,we concluded that cell growth was inhibited in bui1but to a lesser extent at the seedling stage.BUI1Encodes the Class II Formin FH5To understand fully the cellular mechanism by which the bui1mutation inhibits cellular growth,we isolated the BUI1gene using a map-based cloning strategy.An F2population of ;10,000plants was generated from a cross between bui1and Zhenshan 97(an indica cultivar)and was used for PCR-based mapping.The BUI1locus was delimited to a genomic region on chromo-some 7between two simple sequence repeat (SSR)markers,RM1132and RM505(Figure 3A).Six insertion/deletion markers were developed between the two SSR markers,and BUI1was further narrowed down to a 60-kb DNA region between 7WB8and 7WB16on a single BAC,AP004275.There are three open reading frames (ORFs)in this region (Figure 3B).Sequencing analysis revealed that bui1had a substitution of A to G in the sixth intron of the gene FH5/Os07g0596300(Figure 3C),changing the highly conserved 39-intron end AG to GG.We suspected that this mutation interferes with the correct splicing of the sixth intron.Indeed,RT-PCR analysis revealed the presence of the 79-bp intron fragment in the predominantly amplified cDNA fragment of FH5/Os07g0596300(Figure 3D).RNA gel blot analysis was performed to determine the tran-script length of ing a probe specific to its 39region,as supported by an isolated cDNA clone (GenBank accession number AK120222),we detected an ;5-kb-long transcript (Figure 3E),suggesting that the previously predicted full-length mRNA sequence (GenBank accession number NM_001066706)was a truncated one.The detected transcript size is consistent with the predicted protein of the FH5locus (Q84ZL0,isoform 1,annotated by the UniProtKB/Swiss-Prot website),which is 1627amino acids long.The 59region of the FH5transcript was verified by RT-PCR using two sets of primers (Figures 3C and 3F)and sequencing.We thus generated a cDNA clone containing the entire ORF for the annotated FH5polypeptide.This ORF and the native FH5promoter were then cloned into a binary vector,and the resulting transgene was introduced into bui1plants.Figure 3G shows that the FH5minigene successfully complemented the mutant phenotypes.We thus concluded that the A-to-G substi-tution in the FH5locus is responsible for the observed bui1mutant phenotypes.Plant formins are named on the basis of sequence similarity and conservation by phylogenetic analysis,and BUI1corre-sponds to FH5,a class II formin (Cvrckova´et al.,2004;Grunt et al.,2008).FH5is predicted (http://hits.isb-sib.ch/cgi-bin/PFSCAN)to contain a PTEN-like domain (amino acids 198–336)at its N terminus,followed by a typical FH1domain (amino acids 825–1170)and an FH2domain (amino acids 1188–1588;see Supplemental Figure 4A online).The FH1domain consists of 21polyproline stretches (see Supplemental Figure 5online),andFigure parison of Wild-Type and bui1Seedlings.(A)Wild-type (WT)and bui1seedlings (7d old).Note that the bui1shoots are bent.Bar =2cm.(B)to (D)Longitudinal sections of seedling shoots of the wild type (C)and bui1(D).(B)shows 9-d-old seedlings of the wild type (left)and bui1(right).Bar in (B)=2cm;bars in (C)and (D)=100m m.(E)Roots of the wild type (left)and bui1(right)grown in half-strength medium.Bar =1cm.(F)and (G)Cell morphology of wild-type (F)and bui1(G)roots by propidium iodide staining.The regions used for analysis are indicated with squares in (E).Bars =50m m.4of 20The Plant Cellthe FH2domain shares 31,31,29,30,61,and 24%amino acid similarity with those of the formins AFH1,AFH3,At FH5,At FH8,For2A,and Bni1p,respectively (see Supplemental Figure 4B online).Importantly,the key residues (Ile-1431and Lys-1601)of Bni1p involved in actin nucleation and barbed end capping are also conserved in FH5FH2(see Supplemental Figure 4B online;Xu et al.,2004),implying that FH5may perform conserved actin regulatory actions.Inclusion of the sixth intron possibly intro-duced a premature stop codon and could result in a truncated protein that contains the PTEN domain,the FH1domain,and part of the FH2domain in bui1,suggesting that a complete FH2domain is essential for FH5function.Because the mutation in the FH5/BUI1gene is fully recessive,we proposed that the predicted truncated protein,which could accumulate predominantly inbui1,could interfere with the function of the less produced full-length protein.RNA gel blot analysis revealed that FH5is expressed ubiqui-tously in all tissues (Figure 3H).RT-PCR analysis demonstrated that most rice formins are expressed ubiquitously in all the examined tissues,with the exception of FH18and FH12,whose transcripts could be detected only in the young panicles (see Supplemental Figure 6online).Actin Filament Organization in bui1Because formins are known for their abilities to regulate actin cytoskeleton assembly and organization (Goode and Eck,2007),we sought to determine the effect of bui1mutation onactinFigure 3.Map-Based Cloning of BUI1.(A)The BUI1locus was mapped on chromosome 7between two SSR markers,RM1132and RM505.(B)Fine-mapping of BUI1.BUI1was narrowed to a 60-kb region between two makers,7WB8and 7WB16,on a single BAC (AP004275),which contains three predicted genes.(C)Sequence comparison revealed a substitution of A to G in one intron of the gene FH5/Os07g0596300in bui1.WT,wild type.(D)Detection of altered splicing in bui1by RT-PCR analysis.Rice UBI1was used as an internal control.(E)RNA gel blot analysis to confirm the length of the FH5/BUI1transcript.Total RNA (10m g)extracted from the wild type and bui1was used for the analysis (shown below the blot).M,RNA ladder.(F)Confirmation of the full-length FH5/BUI1transcript by RT-PCR.The primer pairs (P1and P2)used for RT are indicated in (C).(G)Complementation test of the FH5/BUI1gene.One representative line (1300-BUI1)of complementation is shown.Bar =2cm.(H)Expression pattern of FH5revealed by RNA gel blot.YS,Young seedling;FL,flower;RA,rachis;LF,leaf;LS,leaf sheath;ND,node;IN,internode;RT,root.Rice FH5Regulates Actin Organization 5of 20organization.We examined the actin cytoskeleton organization in the wild type and bui1directly by staining root cells with AlexaFluor488–phalloidin,which has been widely used for stain-ing actin cytoskeleton in plants.We found that the overall fluorescence signal of the bui1cells was much weaker than that of the wild-type cells under identical staining conditions and confocal settings(Figures4A and4B).Since phalloidin specifi-cally binds to actinfilaments(F-actin),the amount of F-actin is proportional to thefluorescence intensity.Therefore,we decided to compare the F-actin levels between the wild-type and bui1 cells by quantifyingfluorescence intensity.As shown in Figure 4C,the averagefluorescence intensity was reduced by nearly threefold in bui1.These results suggest that FH5/BUI1plays an important role in maintaining the level of F-actin.When the detection settings for bui1were increased so that both the wild-type and bui1cells gave clearfluorescence signals, we found that actinfilaments were severely disorganized in the bui1cells.In wild-type plants,cells in the root elongation zone displayed a clearly organized actin cytoskeleton structure. Prominent actin cables oriented longitudinally through the whole cell cortex and were cross-linked by transversely or longitudi-nally orientedfine actinfilaments(Figure4D).However,longitu-dinal actin cables were barely detected in the bui1cells.Although some thick actin cables were observed in bui1,they were quite short.In addition,thefine actinfilaments were also shorter and arranged randomly in bui1cells compared with those in the wild-type cells(Figure4E).Measurement offluorescence intensity revealed peaks in the wild-type cells,which correspond totheFigure4.F-Actin Organization in Wild-Type and bui1Cells.F-actin organization was visualized by AlexaFluor488–phalloidin staining.Each image is a maximum projection of thefluorescence signals.(A)and(B)F-actin organization in the cortex cells of the root elongation regions of the wild type(A)and bui1(B).Bars=20m m.(C)Quantitative analysis of F-actin levels in wild-type(WT)and bui1cells as detected in(A)and(B).Data shown are means6SE offluorescence intensity of144cells in the wild type and bui1.P<0.01,by t test.(D)and(E)F-actin organization in the root elongation region cells of the wild type(D)and bui1(E).Confocal settings for bui1were increased to give clear signals.Bars=20m m.(G)and(H)F-actin organization in the root transition region cells of the wild type(G)and bui1(H).Confocal settings for bui1were increased to give clear signals.Bars=20m m.(F)and(I)Fluorescence intensities corresponding to the regions marked in(D)/(E)and(G)/(H),respectively.6of20The Plant Cellthick longitudinal actin cables,and such peaks were compro-mised in the bui1cells(Figure4F).We also examined the organization of actin cytoskeleton in the root transition region, where cells undergo rapid elongation.In the wild-type cells,actin cytoskeleton was composed mainly of longitudinal cables that connect with the actin-rich cell ends(Figure4G),which were proposed to support vesicle trafficking and rapid cell expansion (Ketelaar et al.,2003).Such longitudinal actin cables were reduced in the bui1cells,where thin actinfilaments were arranged randomly in the cell cortex(Figure4H).The decrease in the amount of actin cables in bui1root transition region cells was also confirmed by analyzing thefluorescence intensity (Figure4I).Similar results were obtained for the leaf sheath cells (see Supplemental Figure7online).To observe further the actin organization in living cells,we generated a construct with en-hanced greenfluorescent protein(EGFP)fused to both the C and N termini of the actin binding domain2(ABD2)of Arabidopsis fimbrin1(Wang et al.,2008).The resulting EGFP-fABD2-EGFP construct was transformed into wild-type and bui1plants.Both transformations gave more than15independent transgenic lines in which the actinfilaments were parison of actin filament organization in the living cells of these transgenic plants gave similar results to those observed by AlexaFluor488–phal-loidin staining(see Supplemental Figure8online).In summary, mutation of FH5/BUI1resulted in severe disruption of actin filament organization in plant cells.Both FH2and FH1FH2Domains of FH5NucleateActin PolymerizationOur microscopic results showed that FH5is required for the maintenance of F-actin levels and spatial organization of actin filaments in vivo.To investigate the biochemical activities of FH5 on actin cytoskeleton regulation,we attempted to generate recombinant proteins containing the FH2domain and the FH1FH2domain of FH5(see Supplemental Figure4C online). While the FH2domain of FH5was expressed and purified successfully as both a63His fusion and a glutathione S-trans-ferase(GST)fusion(see Supplemental Figure4D,lanes2and3, online),the full-length FH1FH2domain of FH5failed to express in different Escherichia coli strains,yeast cells,or the baculovirus expression system after numerous attempts with varying induc-tion schemes.This might be due to the high content of Pro. Indeed,FH5has21polyproline stretches in the FH1domain, which ranks the most among all rice formins(see Supplemental Figure5online).It was previously shown that the FH1domain is required for formin to bind and elongate actin processively in the presence of profilin and that the number of polyproline stretches in the FH1domain is proportional to the effect of profilin on increasing the elongation rate of barbed ends that are associated with the FH1FH2domain(Kovar and Pollard,2004;Paul and Pollard,2008).Thus,the number of polyproline stretches in a given formin is related quantitatively but not qualitatively to its effect on actin assembly from profilin-bound actin.Therefore,we generated several FH5expression constructs containing FH2 plus the FH1domain with decreasing numbers of polyproline stretches at the N terminus,expressed them in E.coli,and succeeded in expressing and purifying a recombinant FH5FH1FH2protein carrying16polyproline stretches(see Supple-mental Figure4D,lane4,online).Because the nucleation activity is a general and basic feature of formins,wefirst examined the effects of FH5FH1FH2and FH5 FH2on actin polymerization by the pyrene–actin assay and direct visualization of actinfilaments usingfluorescent light micros-copy.In the kinetic pyrene–actin assays,both FH5FH1FH2and FH5FH2recombinant proteins decreased the initial lag phase of actin polymerization in a dose-dependent manner,indicating their active nucleation activity(Figures5A and5B).The FH2 domain alone was sufficient to nucleate actin assembly(Figure 5A).This quality is similar to that of AFH1(Michelot et al.,2005) but is distinct from that of AFH5and AFH3,which nucleate actin assembly only in the presence of the FH1domain(Ingouff et al., 2005;Ye et al.,2009).Direct visualization of actin polymerization showed that addi-tion of100nM FH2or100nM FH1FH2of FH5reduced the length of actinfilaments but increased the number of actinfilaments in the observationfield(see Supplemental Figures9A–9C online), which is in perfect agreement with the pyrene polymerization curves(Figures5A and5B).Similar results were obtained with addition of100nM AFH1FH1FH2(see Supplemental Figure9D online),indicating that these proteins indeed possess actin nucleation activity.Because the length of actinfilaments is known to be inversely proportional to the number of nuclei,we also measured the length of actinfilaments.As shown in Sup-plemental Figure9E online,compared with that of actin alone, the actinfilament lengths were reduced in the presence of100 nM FH5FH2and100nM FH5FH1FH2.Both recombinant proteins behaved quite similarly to the well-characterized AFH1 FH1FH2.These results demonstrated that both FH1FH2and FH2 of FH5could nucleate actin assembly in vitro.FH5Nucleates Actin Assembly from theProfilin/Actin ComplexPrevious studies have shown that in some plant cells,the majority of actin monomers are sequestered by profilin,a highly abundant actin binding protein,to suppress spontaneous actin polymerization(Gibbon et al.,1997;Wang et al.,2005;Chaudhry et al.,2007).Several Arabidopsis formins have been shown to nucleate actin assembly from the profilin/actin complex(Deeks et al.,2005;Ingouff et al.,2005;Michelot et al.,2005;Yi et al., 2005;Ye et al.,2009).We thus decided to test whether FH5also possesses such a nucleation activity.As shown in Figure5D,FH5 FH2nucleated actin efficiently in the absence of profilin.How-ever,when adding an equimolar amount of human profilin I,the initial lag time corresponding to the nucleation phase increased, suggesting that profilin strongly inhibited the FH5FH2–facilitated actin nucleation.By contrast,FH5FH1FH2could reduce the initial lag phase,suggesting active nucleation(Figure5E).We thus concluded that FH5is capable of nucleating actin assembly from the profilin/actin complex,which absolutely requires the polyproline-rich FH1domain.We further analyzed the effect of FH5on actin assembly from the profilin/actin complex by directly visualizing actin assembly via total internal reflectionfluorescence microscopy(TIRFM). Actin polymerization was observed near the surface of the cover Rice FH5Regulates Actin Organization7of20。
plant cell 2012 chen rong
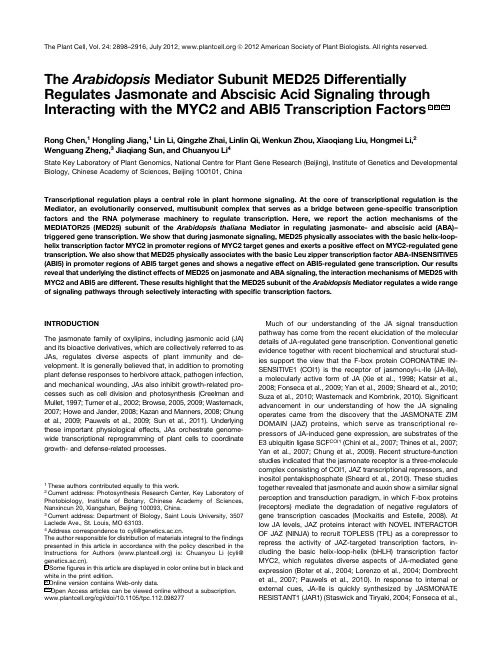
The Arabidopsis Mediator Subunit MED25Differentially Regulates Jasmonate and Abscisic Acid Signaling through Interacting with the MYC2and ABI5Transcription Factors C W OA Rong Chen,1Hongling Jiang,1Lin Li,Qingzhe Zhai,Linlin Qi,Wenkun Zhou,Xiaoqiang Liu,Hongmei Li,2 Wenguang Zheng,3Jiaqiang Sun,and Chuanyou Li4State Key Laboratory of Plant Genomics,National Centre for Plant Gene Research(Beijing),Institute of Genetics and Developmental Biology,Chinese Academy of Sciences,Beijing100101,ChinaTranscriptional regulation plays a central role in plant hormone signaling.At the core of transcriptional regulation is the Mediator,an evolutionarily conserved,multisubunit complex that serves as a bridge between gene-specific transcription factors and the RNA polymerase machinery to regulate transcription.Here,we report the action mechanisms of the MEDIATOR25(MED25)subunit of the Arabidopsis thaliana Mediator in regulating jasmonate-and abscisic acid(ABA)–triggered gene transcription.We show that during jasmonate signaling,MED25physically associates with the basic helix-loop-helix transcription factor MYC2in promoter regions of MYC2target genes and exerts a positive effect on MYC2-regulated gene transcription.We also show that MED25physically associates with the basic Leu zipper transcription factor ABA-INSENSITIVE5 (ABI5)in promoter regions of ABI5target genes and shows a negative effect on ABI5-regulated gene transcription.Our results reveal that underlying the distinct effects of MED25on jasmonate and ABA signaling,the interaction mechanisms of MED25with MYC2and ABI5are different.These results highlight that the MED25subunit of the Arabidopsis Mediator regulates a wide range of signaling pathways through selectively interacting with specific transcription factors.INTRODUCTIONThe jasmonate family of oxylipins,including jasmonic acid(JA) and its bioactive derivatives,which are collectively referred to as JAs,regulates diverse aspects of plant immunity and de-velopment.It is generally believed that,in addition to promoting plant defense responses to herbivore attack,pathogen infection, and mechanical wounding,JAs also inhibit growth-related pro-cesses such as cell division and photosynthesis(Creelman and Mullet,1997;Turner et al.,2002;Browse,2005,2009;Wasternack, 2007;Howe and Jander,2008;Kazan and Manners,2008;Chung et al.,2009;Pauwels et al.,2009;Sun et al.,2011).Underlying these important physiological effects,JAs orchestrate genome-wide transcriptional reprogramming of plant cells to coordinate growth-and defense-related processes.Much of our understanding of the JA signal transduction pathway has come from the recent elucidation of the molecular details of JA-regulated gene transcription.Conventional genetic evidence together with recent biochemical and structural stud-ies support the view that the F-box protein CORONATINE IN-SENSITIVE1(COI1)is the receptor of jasmonoyl-L-Ile(JA-Ile), a molecularly active form of JA(Xie et al.,1998;Katsir et al., 2008;Fonseca et al.,2009;Yan et al.,2009;Sheard et al.,2010; Suza et al.,2010;Wasternack and Kombrink,2010).Significant advancement in our understanding of how the JA signaling operates came from the discovery that the JASMONATE ZIM DOMAIN(JAZ)proteins,which serve as transcriptional re-pressors of JA-induced gene expression,are substrates of the E3ubiquitin ligase SCF COI1(Chini et al.,2007;Thines et al.,2007; Yan et al.,2007;Chung et al.,2009).Recent structure-function studies indicated that the jasmonate receptor is a three-molecule complex consisting of COI1,JAZ transcriptional repressors,and inositol pentakisphosphate(Sheard et al.,2010).These studies together revealed that jasmonate and auxin show a similar signal perception and transduction paradigm,in which F-box proteins (receptors)mediate the degradation of negative regulators of gene transcription cascades(Mockaitis and Estelle,2008).At low JA levels,JAZ proteins interact with NOVEL INTERACTOR OF JAZ(NINJA)to recruit TOPLESS(TPL)as a corepressor to repress the activity of JAZ-targeted transcription factors,in-cluding the basic helix-loop-helix(bHLH)transcription factor MYC2,which regulates diverse aspects of JA-mediated gene expression(Boter et al.,2004;Lorenzo et al.,2004;Dombrecht et al.,2007;Pauwels et al.,2010).In response to internal or external cues,JA-Ile is quickly synthesized by JASMONATE RESISTANT1(JAR1)(Staswick and Tiryaki,2004;Fonseca et al.,1These authors contributed equally to this work.2Current address:Photosynthesis Research Center,Key Laboratory ofPhotobiology,Institute of Botany,Chinese Academy of Sciences,Nanxincun20,Xiangshan,Beijing100093,China.3Current address:Department of Biology,Saint Louis University,3507Laclede Ave.,St.Louis,MO63103.4Address correspondence to cyli@.The author responsible for distribution of materials integral to thefindingspresented in this article in accordance with the policy described in theInstructions for Authors()is:Chuanyou Li(cyli@).C Somefigures in this article are displayed in color online but in black andwhite in the print edition.W Online version contains Web-only data.OA Open Access articles can be viewed online without a subscription./cgi/doi/10.1105/tpc.112.098277The Plant Cell,Vol.24:2898–2916,July2012,ã2012American Society of Plant Biologists.All rights reserved.2009;Suza et al.,2010;Wasternack and Kombrink,2010).JA-Ile then acts as“molecular glue”to stimulate the interaction of its coreceptors COI1and JAZs and,as a consequence,promotes the degradation of the JAZ repressors.Degradation of JAZ proteins leads to the liberation of MYC2from NINJA and TPL and initiates the transcriptional reprogramming of the cells and the activation of the JA responses(Chini et al.,2007;Thines et al.,2007;Pauwels et al.,2010;Sheard et al.,2010).In ad-dition to MYC2,several other transcription factors targeted by JAZs were recently identified.Among them,MYC3and MYC4 act additively with MYC2in the regulation of root growth in-hibition and defense responses(Fernández-Calvo et al.,2011). The WD-repeat/bHLH/MYB transcription factor complexes TRANSPARENT TESTA8/GLABRA3(GL3)/ENHANCER OF GL3/MYB75/GL1are involved in JA-induced anthocyanin accumulation and trichome initiation(Qi et al.,2011).The R2R3-MYB transcription factors MYB21/MYB24are specifi-cally involved in JA-dependent anther development(Song et al.,2011).Emerging evidence has revealed the involvement of the Me-diator complex in JA-induced transcriptional regulation(Kidd et al.,2009).Mediator is a conserved multisubunit complex known to promote the transcription of protein-coding genes by RNA polymerase II(Pol II)in eukaryotes.Mediator wasfirst identified from yeast as required for activator-dependent stim-ulation of Pol II transcription(Kelleher et al.,1990;Flanagan et al.,1991).Later it was shown that the yeast Mediator is a multiprotein complex that forms three submodules,the head, middle,and tail,with the head submodule serving as the Pol II–interacting interface and the tail submodule interacting with sequence-specific transcription factors(Chadick and Asturias, 2005).It was now realized that Mediator is highly conserved in a wide range of eukaryotes,including mammals and plants (Chadick and Asturias,2005;Malik and Roeder,2005;Bäckström et al.,2007).The studies over the last decade reveal that,besides interacting directly with Pol II,Mediator has multiple functions and can interact with and coordinate the action of numerous other coactivators and corepressors,including those acting at the level of chromatin(Kidd et al.,2010,2011;Malik and Roeder,2010; Borggrefe and Yue,2011;Chen and Roeder,2011;Conaway and Conaway,2011;Hentges,2011;Kim and Chen,2011;Ries and Meisterernst,2011).The recent purification of the Arabidopsis thaliana Mediator complex identified21conserved and six plant-specific subunits (Bäckström et al.,2007).Among the few genetically character-ized Arabidopsis Mediator subunits(Autran et al.,2002;Wang and Chen,2004;Gillmor et al.,2010;Ito et al.,2011;Kidd et al., 2011;Kim et al.,2011),MEDIATOR25(MED25)was shown to play multiple roles in regulatingflowering(Cerdán and Chory, 2003),organ size(Xu and Li,2011),stress responses(Elfving et al.,2011),and,notably,JA signaling(Kidd et al.,2009).These studies suggest that a single Mediator subunit can serve as the interface for several diverse transcriptional regulators and raise a significant question of how a high degree of plasticity of a single Mediator subunit is achieved.Here,we investigate the distinct action mechanisms of MED25in regulating JA-and abscisic acid(ABA)–induced gene expression.We show that MED25interacts with the bHLH transcription factor MYC2and executes a positive effect on MYC2-regulated expression of JA-responsive genes.We also show that MED25interacts with the basic domain/Leu zipper (bZIP)transcription factor ABA-INSENSITIVE5(ABI5)and exerts a negative effect on ABI5-regulated expression of ABA-re-sponsive genes.We provide evidence showing that the con-served activator-interacting domain(ACID)of MED25is sufficient for its interaction with ABI5but not sufficient for its interaction with MYC2.In addition,we reveal that while the putative tran-scriptional activation domain(TAD)of MYC2is involved in its in-teraction with MED25,the TAD domain of ABI5is not involved in its interaction with MED25.Thesefindings highlight that,in re-sponse to different hormonal signals,a single plant Mediator subunit can regulate distinct transcriptional programs via inter-actions with relevant gene-specific transcription factors. RESULTSThe ber6Mutant Contains a Mutation in the MED25Gene and Affects Diverse Aspects of JA ResponsesThe bestatin-resistant6(ber6)line of Arabidopsis was identified as a JA-insensitive mutant in JA-induced root growth inhibition (Zheng et al.,2006).Our further characterization of ber6was performed with a homozygous line obtained fromfive suc-cessive backcrosses of the original mutant to the wild type Columbia-0(Col-0).JA response assays with this line showed that ber6was generally less sensitive than the wild type to the in-hibition effect of a range of concentrations of JA on root growth (Figure1A).We then examined whether the expression of JA-responsive genes is affected in ber6plants.Two distinct branches of JA-responsive genes that are differentially regulated by the transcription factor MYC2were identified in Arabidopsis (Boter et al.,2004;Lorenzo et al.,2004).One of these branches, including the VEGETATIVE STORAGE PROTEIN1(VSP1)gene (Berger et al.,1995),is believed to be involved in plant re-sponses to wounding.The other branch,including the plant defensin gene PDF1.2(Penninckx et al.,1996),is considered to be associated with plant responses to pathogen infection(Boter et al.,2004;Lorenzo et al.,2004).Promoter-reporter fusion as-says revealed that the ber6mutation substantially impaired JA-induced expression of both VSP1pro:GUS(for b-glucuronidase)and PDF1.2pro:LUC(for luciferase)(Figures1B and1C).Con-sistent with this,quantitative real-time PCR(qRT-PCR)assays showed that the JA-induced expression levels of VSP1(Figure 1D)and PDF1.2(Figure1E)were markedly reduced in ber6 compared with those in the wild type.Next,we examined the response of ber6plants to infection by Pseudomonas syringae pv tomato(Pst)DC3000.As measured by leaf symptoms(Figure 1F)and pathogen growth(Figure1G)of the infected plants, the effects of Pst DC3000infection in ber6are largely similar to those in the coi1-2mutant,which harbors a point mutation of the JA receptor gene COI1and therefore is resistant to Pst DC3000(Kloek et al.,2001;Xu et al.,2002;Laurie-Berry et al.,2006).Together,our results support that ber6is a JA signaling mutant that is defective in JA-induced defense gene expression.MED25Regulates JA and ABA Signaling2899Figure 1.The Defective JA Responses of the ber6Mutant Are Caused by a Mutation of the MED25Gene.(A)Root growth inhibition assay of 10-d-old seedlings from Col-0and ber6grown in MS medium containing indicated concentrations of JA.Results shown are the mean 6SD of measurements from 90seedlings.For each JA concentration,the data were analyzed by Student ’s t test using SPSS.Asterisks denote t test signi ficance compared with JA-grown wild type plants:**P <0.01.(B)Photographs of representative control and 50m M MeJA (6-h treatments)treated 10-d-old seedlings of VSP1pro :GUS/Col-0and VSP1pro :GUS/ber6.(C)Photographs of representative control and 50m M MeJA (48-h treatments)treated 10-d-old seedlings of PDF1.2pro :LUC/Col-0and PDF1.2pro :LUC/ber6.(D)and (E)qRT-PCR analysis of VSP1(6-h treatments;[D])and PDF1.2(48-h treatments;[E])RNA levels in 10-d-old Col-0and ber6seedlings treated with 50µM MeJA.The break ranges are shown on the y axis.Data shown are mean values of three biological repeats with SD .Asterisks denote Student ’s t test signi ficance compared with MeJA-treated Col-0plants:**P <0.01.Numbers on the white bars represent fold induction values in MeJA-treated plants relative to control (black bars)plants.(F)Symptoms on rosette leaves of 4-week-old soil-grown Col-0,ber6,and coi1-2following inoculation with Pst DC3000bacteria for 3d.(G)Pst DC3000populations,shown as colony-forming units (c.f.u.)cm 22of leaf area in Col-0,ber6,and coi1-2plants at day 0(black)and day 3(white)after inoculation.Asterisks denote Student ’s t test signi ficance compared with Pst DC3000–treated Col-0plants:**P <0.01.(H)Fine genetic and physical mapping of BER6.The target gene was mapped to a genetic interval between markers F21J9and T24P13on chro-mosome 1.Analysis of a F2mapping population of 1800plants delimited the gene to a region of 50kb on the BAC clone F2J7(red).Numbers in parentheses indicate the number of recombination events identi fied between markers and the target gene.(I)MED25gene structure,showing the mutation site of ber6/med25-4.The T-DNA insertion sites in med25-2,med25-3,pft1-1,and pft1-2and the point mutation site of med25-1are also indicated.Black boxes represent exons,lines represent introns,and white boxes represent 59(39)-untranslated region,respectively.Bar =1kb.(J)Schematic representation of MED25protein.The MED25protein contains a vWF-A domain (blue),an MD domain (white),an ACID domain (red),and a GD domain (gray).Bar =100amino acids (aa).The decreased JA-induced root growth inhibition of ber6 provides a facile assay for map-based cloning studies aimed at determining the genetic basis of this ing a total of 1800F2plants showing the mutant phenotype,BER6was de-limited to a50-kb region on the BAC clone F2J7(Figure1H). DNA sequencing revealed that ber6contains a G-to-A transition at the junction between thefifth exon and thefifth intron of the annotated gene At1g25540(Figure1I),which encodes the MED25subunit of the Arabidopsis Mediator(Bäckström et al., 2007).The G-to-A mutation in ber6destroys an Rsa I restriction site,and a cleaved amplified polymorphic sequence marker was developed to detect the ber6mutant allele(see Supplemental Figure1C online).In the ber6mutant,the59exon-intron boundary of intron5is changed from GT to AT(Figure1I),re-placing a G that is highly conserved at plant gene splice sites. The mutation in ber6altered the splicing of At1g25540mRNA (see Supplemental Figure1A online),resulting in a frame shift that destroys the von Willebrand Factor A(vWF-A)domain, which has been shown to be crucial for the binding of MED25to the Mediator complex(Mittler et al.,2003).Protein gel analysis using anti-MED25antibodies failed to detect MED25protein accumulation in the ber6mutant(see Supplemental Figure1B online).For genetic complementation of the ber6phenotype,the MED25cDNA from the wild type was introduced into ber6 mutants under the control of the35S promoter of Cauliflower mosaic virus.The results showed that the MED25cDNA com-plemented the ber6mutant in JA-mediated root growth in-hibition(see Supplemental Figure1C online).These results support that the defective JA response phenotype of ber6is resulted from the identified G-to-A mutation of MED25.Since several mutant alleles of the MED25gene have been described (Cerdán and Chory,2003;Xu and Li,2011),we henceforth designated the ber6allele as med25-4.Further confirmation that BER6encodes MED25came from analyses of several reported mutant alleles of MED25(Figure1I).pft1-1,the original mutant allele of MED25(Cerdán and Chory,2003),as well as the re-cently described alleles,including pft1-2(Kidd et al.,2009), med25-1,med25-2,and med25-3(Xu and Li,2011),all dis-played reduced sensitivity to JA in root growth inhibition(see Supplemental Figure1E online).Genetic analyses showed that ber6/med25-4and pft1-2cannot complement each other in JA-induced root growth inhibition(see Supplemental Figure1F online). In addition,ber6/med25-4displayed a lateflowering phenotype when grown under long-day conditions(16h light/8h dark),as de-termined by rosette leaf number and bolting time(see Supplemental Figure1G online).When grown under short-day conditions(10h light/14h dark),this mutant had similar rosette leaf number as the wild type but the bolting time showed a significant increase(see Supplemental Figure1H online).Similar lateflowering phenotype has also been observed in most of the reported mutant alleles of MED25 (Cerdán and Chory,2003;Kidd et al.,2009).Collectively,these results demonstrate that the med25mutation we identified is responsible for the ber6mutant phenotype.The MED25protein contains a vWF-A domain(MED251-226), a nonconserved middle domain(MD;MED25227-550),an ACID domain(MED25551-680),and a Gln-rich domain(GD;MED25681-836) (Figure1J).It is generally believed that the vWF-A domain is es-sential for the binding of MED25to the Mediator complex,and the ACID domain is important for interaction with transcriptional acti-vators,whereas the GD domain could be important for transcrip-tional activation(Cerdán and Chory,2003;Mittler et al.,2003; Bäckström et al.,2007;Lee et al.,2007;Elfving et al.,2011). MED25Affects the Function of MYC2in Regulating the Transcriptional Expression of JA-Responsive GenesTo elucidate the action mechanisms of MED25in JA-mediated transcriptional regulation,we investigated the effect of MED25 on the function of MYC2,a master transcriptional regulator that controls diverse aspects of JA responses(Boter et al.,2004; Lorenzo et al.,2004;Dombrecht et al.,2007).Toward this goal, we generated a double mutant line med25-4myc2-2and compared its JA responses with those of the single mutants in defense gene expression.Consistent with the well-recognized finding that MYC2negatively regulates the expression of the branch of pathogen-responsive genes(Boter et al.,2004;Lorenzo et al.,2004;Dombrecht et al.,2007),our qRT-PCR assays in-dicated that JA-induced expression levels of PDF1.2(Figure2A) and ETHYLENE RESPONSE FACTOR1(ERF1;Figure2B)were markedly increased in myc2-2compared with those in the wild type.Importantly,JA-induced expression levels of these genes were strongly reduced in the med25-4mutant(Figures1E,2A, and2B),suggesting that MED25is required for JA-induced expression of this group of genes.Significantly,in the med25-4 myc2-2double mutant,JA-induced expression levels of PDF1.2 and ERF1were essentially comparable to those in med25-4(i.e., med25-4suppressed the phenotype of myc2-2in terms of JA-induced PDF1.2and ERF1expression)(Figures2A and2B), suggesting that MED25acts genetically downstream of MYC2in regulating the expression of these genes.Together,these results support that,in response to JA,MED25is required for the MYC2 function to repress the expression of pathogen-responsive genes. Consistent with the positive role of MYC2in regulating the ex-pression of wound-responsive genes(Boter et al.,2004;Lorenzo et al.,2004;Dombrecht et al.,2007),we found that the JA-induced expression levels of VSP1(Figure2C),LIPOXYGENASE2 (LOX2;Figure2D),JAZ6(Figure2E),and JAZ8(Figure2F)were substantially reduced in myc2-2mutants compared with those in wild-type plants(Figures2C to2F).JA-induced expression levels of these genes were also reduced in med25-4(Figures2C to2F), suggesting that MED25is also important for JA-induced ex-pression of this group of genes.As expected,in the med25-4 myc2-2double mutant,JA-induced expression levels of these genes were largely similar to those in med25-4(Figures2C to2F), suggesting that MED25is also important for the MYC2function to activate the expression of these wound-responsive genes.Taken together,these results led us to the conclusion that,during JA response,MED25exerts a positive effect on the function of the MYC2transcription factor to regulate the expression of JA-responsive genes.MED25Is Recruited to Promoters of MYC2Target Genes in a JA-Dependent MannerIt has been shown that the human MED25,together with the retinoic acid(RA)–bound retinoic acid receptor(RAR),wasMED25Regulates JA and ABA Signaling2901recruited to the promoter regions of RA-responsive genes and therefore regulates the function of RAR (Lee et al.,2007).In this context,we speculate that the Arabidopsis MED25could associ-ate with MYC2in chromatin regions of MYC2target genes.To test this hypothesis,we first assessed the effect of MED25on the expression JA-responsive genes that are directly targeted by MYC2.It has been shown that in response to JA,MYC2quickly activates the expression of the JAZ family genes via directly binding to the G-box (and T/G-box)sequence in the promoter of these genes (Chini et al.,2007;Hou et al.,2010).Our qRT-PCR assays revealed that the med25-4mutation markedly reduced the JA-induced expression levels of the JAZ family genes (see Supplemental Figure 2online).Chromatin immunoprecipitation(ChIP)assays using 35S pro :MYC2-GFP (for green fluorescent protein)plants and anti-GFP antibodies indicated that MYC2as-sociated with the G-box (59-CACGTG-39)motifs in the promoters of JAZ6and JAZ8(Figures 3A and 3B),con firming that JAZ6and JAZ8are direct targets of MYC2.ChIP assays using 35S pro :MED25-GFP plants indicated that,like MYC2,MED25was also enriched in the G-box (59-CACGTG-39)motifs of JAZ6and JAZ8promoters,and,importantly,the enrichment of both MYC2and MED25was substantially enhanced by JA treatment (Figure 3B).These results demonstrate that MED25associates with the tran-scription factor MYC2in chromatin regions of MYC2target genes.To determine the recruitment dynamic of MYC2and MED25to JAZ promoters,35S pro :MYC2-GFP and 35S pro :MED25-GFPFigure 2.MED25Affects the Function of MYC2in Regulating the Transcriptional Expression of JA-Responsive Genes.Expression of PDF1.2(48-h treatments;[A]),ERF1(1-h treatments;[B]),VSP1(6-h treatments;[C]),LOX2(1-h treatments;[D]),JAZ6(1-h treatments;[E]),and JAZ8(1-h treatments;[F])was examined by qRT-PCR in Col-0,med25-4,myc2-2,and med25-4myc2-2plants following 50µM MeJA treatment.The break ranges (for PDF1.2,VSP1,and JAZ8)are shown on the y axis.Data presented are mean values of three biological repeats with SD .Numbers on the white bars represent fold induction values in MeJA-treated plants relative to control (black bars)plants for each genotype.2902The Plant Cellplants were incubated with JA for varying lengths of time.ChIP assays indicated that low levels of MYC2was recruited to JAZ6promoter at steady state and that JA treatment leads to a marked increase of MYC2recruitment within 15min;MYC2binding to JAZ6promoter then remains at high levels during the time course investigated (Figure 3C).Parallel experiments re-vealed that MED25shows negligible association with JAZ6promoter at steady state (Figure 3D).In the presence of the li-gand,however,MED25recruitment was quickly increased and reached a maximum within 15min after JA treatment (Figure 3D);MED25binding then exhibited a tendency of reduction for the duration of the experiment (Figure 3D).Next,we examined whether the JAZ6mRNA levels follow the recruitment pattern of MED25to the promoter of JAZ6.As shown in Figure 3E,in wild-type plants,JAZ6mRNA levels increased markedly at 15min,peaked at 60min,and then showed a tendency of decrease up to 180min upon JA treatment.In JA-treated med25-4plants,JAZ6expression exhibited similar induction kinetics,albeit its expres-sion levels are substantially reduced in med25-4compared with those in wild-type plants (Figure 3E).These results indicate that the maximum recruitment of MED25to the JAZ6promoter occurs generally earlier than the peaked activation of JAZ6expression.This scenario is consistent with a previous observation in mam-malian system showing that Mediator and other coactivatorsareFigure 3.MED25Is Recruited to Promoter Regions of MYC2Target Genes in a JA-Dependent Manner.(A)Schematic diagram of the promoter region of JAZ6and JAZ8.Black lines represent the promoter region of the two genes.Black boxes on the line represent the putative MYC2binding G-box (59-CACGTG-39);numbers above indicate the distance away from the ATG.Region between the two coupled arrowheads indicates the DNA fragments used for ChIP-PCR.The translational start sites (ATG)were shown as +1.Bar =200bp.(B)Binding of MYC2and MED25to JAZ6and JAZ8promoter regions.The 35S pro :MYC2-GFP and 35S pro :MED25-GFP transgenic seedlings were used in ChIP using anti-GFP antibody (Abcam).For MeJA treatment,35S pro :MYC2-GFP and 35S pro :MED25-GFP seedlings were treated with 100µM MeJA for 60min before cross-linking.The “No Ab ”(no antibody)immunoprecipitates served as negative controls.The ChIP signal was quanti fied as the percentage of total input DNA by real-time PCR.Three biological replicates were performed and identical results were obtained.Standard deviations were calculated from three technical repeats.(C)and (D)Dynamic recruitment of MYC2and MED25to JAZ6promoter.ChIP assays were performed as in (B),except that 35S pro :MYC2-GFP and 35S pro :MED25-GFP plants were treated with 100µM MeJA for varying lengths of time (0,15,60,and 180min)before cross-linking.(E)JAZ6mRNA level follows the recruitment pattern of MED25to the promoter.Col-0and med25-4were treated with MeJA for varying lengths of time (0,15,60,and 180min)as in (C)and (D).Data presented are mean values of three biological repeats with SD .[See online article for color version of this figure.]MED25Regulates JA and ABA Signaling 2903targeted to a promoter before significant gene activation occurs (Sharma and Fondell,2002).Mapping of the Domains Involved in the MED25/MYC2InteractionIt has been shown that the MED25counterparts from animals physically associate with multiple cellular transcriptional activa-tors,including the herpes simplex viral activator VP16(Mittler et al.,2003;Yang et al.,2004),RAR(Lee et al.,2007),hepatocyte nuclear factor4(Rana et al.,2011),and the chondrogenesis-related transcriptional activator Sox9(Nakamura et al.,2011).Our data showing that mutation of the Arabidopsis MED25gene affects the action of MYC2in regulating the expression of JA-responsive genes prompted us to characterize the physical interaction of the two proteins in vivo.Toward this goal,we conducted coimmu-noprecipitation(Co-IP)experiments using our recently described 35Spro:MYC2-4myc plants(Chen et al.,2011)and anti-MED25 antibody.Co-IP assays revealed that,in the absence of exoge-nous JA,MED25could be precipitated by MYC2(Figure4A), suggesting that MED25interacts with MYC2at steady state. Parallel Co-IP assays indicated that the precipitation efficiency of MED25by MYC2was not substantially changed by JA treatment for30min(Figure4A),suggesting that JA shows little effect on the MED25and MYC2interaction at the time point investigated.Next,we tried to map the interaction domain of MED25with MYC2using yeast two-hybrid assays.For these experiments,we fused the full length or derivatives of MED25to the GAL4-DNA binding domain and fused the full-length MYC2 to the GAL4-activation domain(Figure4B).To circumvent the problem that full-length MED25can activate the transcription of reporter genes in yeast(Ou et al.,2011),we performed our yeast two-hybrid assays in the presence of10mM3-amino1,2,4-triazol (Bharti et al.,2000),which could effectively repress the back-ground transcription activation activity of full-length MED25(see Supplemental Figure3online).Under these experimental con-ditions,we found that full-length MED25showed interaction with MYC2in yeast(Figure4B;see Supplemental Figure3online). On the contrary,none of the four individual MED25domains, including the vWF-A(MED25vWF-A),the MD(MED25MD),the ACID(MED25ACID)and the GD(MED25GD)domains,showed interaction with MYC2in yeast(Figure4B).In the context that the ACID domain serves as the ACID of Arabidopsis MED25 (Elfving et al.,2011;Ou et al.,2011)as well as its mammalian counterparts(Mittler et al.,2003;Yang et al.,2004;Lee et al., 2007)and that deletion of the ACID and MD,but not of the vWF-A and GD,destroyed MED25interaction with MYC2(Fig-ure4B),we propose that the ACID and the MD are important for MED25interaction with MYC2.Indeed,the MD-ACID fusion (MED25MD-ACID)showed interaction with MYC2in yeast two-hybrid assays(Figure4B).We then employed a yellowfluorescent protein(YFP)bimolecularfluorescence complementation(BiFC) system(Weinthal and Tzfira,2009)to characterize further the MYC2-interacting domain of MED25.Like the full-length MED25 (Figure4C),the MD-ACID fusion(MED25MD-ACID)showed in-teraction with MYC2in BiFC assays(Figure4D).On the contrary, neither MED25MD(Figure4E)nor MED25ACID(Figure4F)alone showed interaction with MYC2.Further mapping efforts using BiFC assays revealed that the smallest positive MED25fragment (MED25540-680)included the ACID domain plus10amino acids of the MD(Figure4G).Together,these data indicate that the ACID domain alone is not sufficient for MED25interaction with MYC2. We then use yeast two-hybrid assays to map the domain of MYC2responsible for interaction with MED25.For these experiments,several MYC2protein derivatives were fused to the GAL4-activation domain and full-length MED25was fused to the GAL4-DNA binding domain(Figure4H).As indicated in Figure 4H,the N-terminal part(MYC21-188)containing the putative TAD (MYC2149-188)(Lorenzo et al.,2004;Fernández-Calvo et al., 2011)showed interaction with MED25,whereas no interaction was observed using the middle part(MYC2189-445)or C-terminal part(MYC2446-624).Moreover,the putative TAD of MYC2alone was sufficient to interact with MED25(Figure4H).It has been shown that in yeast and animals,the Mediator complex physically associates with both DNA-bound tran-scription factors and Pol II and thus serves as an integrative hub for transcriptional regulation(Malik and Roeder,2005,2010; Conaway and Conaway,2011;Larivière et al.,2012).In this context,our results showing that the Arabidopsis MED25physi-cally associates with MYC2and strongly affects its function sug-gests that mutation of MED25could impair the recruitment of the general transcriptional machinery during JA-triggered gene tran-scription.To test this,we assessed the effect of the med25-4 mutation on JA-induced recruitment of NRPB2(DNA-directed RNA polymerase II subunit RPB2),the second largest subunit of Pol II (Onodera et al.,2005,2008;Zheng et al.,2009),to the promoter of MYC2target genes.Indeed,ChIP assays revealed that JA-induced recruitment of NRPB2to JAZ6promoter was markedly reduced in med25-4compared with that in the wild type(Figure4I).In a current view of MYC2-directed transcription of JA-responsive genes,it is hypothesized that the JAZ-mediated repression of MYC2function is achieved through recruitment of the corepressor TPL by NINJA,which physically associates with JAZs(Pauwels et al.,2010).This scenario predicts that during JA response,both MED25and TPL could be recruited to the promoter of MYC2targets.Indeed,our ChIP assays revealed that,as with MYC2(Figures3B and3C)and MED25(Figures3B and3D),the TPL corepressor was also recruited to the JAZ6promoter(Figure 4J).Importantly,TPL recruitment to JAZ6promoter was sub-stantially reduced upon1h of JA treatment(Figure4J),suggesting that TPL recruitment to MYC2target promoters is regulated by the JA ligand.In addition,Co-IP assays using the reported35Spro:TPL-GFP plants(Szemenyei et al.,2008)and anti-MED25antibody in-dicated that MED25and TPL could exist in a protein complex (Figure4K),albeit yeast two-hybrid assays failed to detect their direct physical interaction(see Supplemental Figure4online). Taken together,these data support that MED25may act as part of the general transcriptional machinery in regulating JA-triggered gene expression.MED25Negatively Regulates ABA Response during Seed Germination and Early Seedling GrowthConsidering that MED25regulates plant responses to a variety of abiotic stresses(Elfving et al.,2011),in which the phytohor-mone ABA plays an important regulatory role,we asked whether2904The Plant Cell。
Supply Route_ ABCG Transporters Act in the Construction of Suberin Barriers
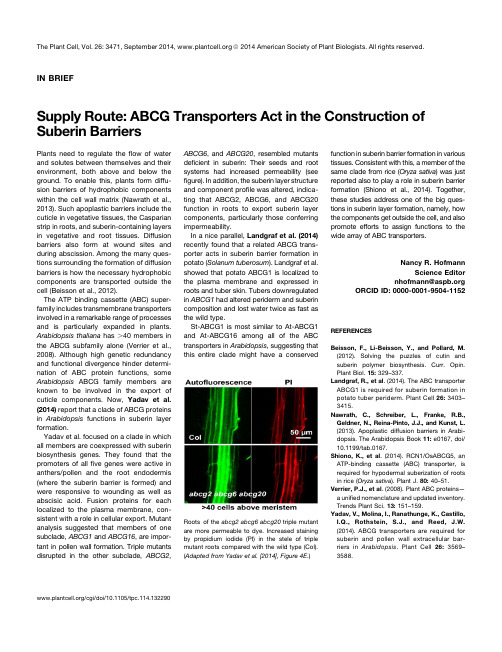
IN BRIEFSupply Route:ABCG Transporters Act in the Construction of Suberin BarriersPlants need to regulate theflow of water and solutes between themselves and their environment,both above and below the ground.To enable this,plants form diffu-sion barriers of hydrophobic components within the cell wall matrix(Nawrath et al., 2013).Such apoplastic barriers include the cuticle in vegetative tissues,the Casparian strip in roots,and suberin-containing layers in vegetative and root tissues.Diffusion barriers also form at wound sites and during abscission.Among the many ques-tions surrounding the formation of diffusion barriers is how the necessary hydrophobic components are transported outside the cell(Beisson et al.,2012).The ATP binding cassette(ABC)super-family includes transmembrane transporters involved in a remarkable range of processes and is particularly expanded in plants. Arabidopsis thaliana has.40members in the ABCG subfamily alone(Verrier et al., 2008).Although high genetic redundancy and functional divergence hinder determi-nation of ABC protein functions,some Arabidopsis ABCG family members are known to be involved in the export of cuticle components.Now,Yadav et al. (2014)report that a clade of ABCG proteins in Arabidopsis functions in suberin layer formation.Yadav et al.focused on a clade in which all members are coexpressed with suberin biosynthesis genes.They found that the promoters of allfive genes were active in anthers/pollen and the root endodermis (where the suberin barrier is formed)and were responsive to wounding as well as abscisic acid.Fusion proteins for each localized to the plasma membrane,con-sistent with a role in cellular export.Mutant analysis suggested that members of one subclade,ABCG1and ABCG16,are impor-tant in pollen wall formation.Triple mutants disrupted in the other subclade,ABCG2,ABCG6,and ABCG20,resembled mutantsdeficient in suberin:Their seeds and rootsystems had increased permeability(seefigure).In addition,the suberin layer structureand component profile was altered,indica-ting that ABCG2,ABCG6,and ABCG20function in roots to export suberin layercomponents,particularly those conferringimpermeability.In a nice parallel,Landgraf et al.(2014)recently found that a related ABCG trans-porter acts in suberin barrier formation inpotato(Solanum tuberosum).Landgraf et al.showed that potato ABCG1is localized tothe plasma membrane and expressed inroots and tuber skin.Tubers downregulatedin ABCG1had altered periderm and suberincomposition and lost water twice as fast asthe wild type.St-ABCG1is most similar to At-ABCG1and At-ABCG16among all of the ABCtransporters in Arabidopsis,suggesting thatthis entire clade might have a conservedfunction in suberin barrier formation in varioustissues.Consistent with this,a member of thesame clade from rice(Oryza sativa)was justreported also to play a role in suberin barrierformation(Shiono et al.,2014).Together,these studies address one of the big ques-tions in suberin layer formation,namely,howthe components get outside the cell,and alsopromote efforts to assign functions to thewide array of ABC transporters.Nancy R.HofmannScience Editornhofmann@ORCID ID:0000-0001-9504-1152REFERENCESBeisson,F.,Li-Beisson,Y.,and Pollard,M.(2012).Solving the puzzles of cutin andsuberin polymer biosynthesis.Curr.Opin.Plant Biol.15:329–337.Landgraf,R.,et al.(2014).The ABC transporterABCG1is required for suberin formation inpotato tuber periderm.Plant Cell26:3403–3415.Nawrath, C.,Schreiber,L.,Franke,R.B.,Geldner,N.,Reina-Pinto,J.J.,and Kunst,L.(2013).Apoplastic diffusion barriers in Arabi-dopsis.The Arabidopsis Book11:e0167,doi/10.1199/tab.0167.Shiono,K.,et al.(2014).RCN1/OsABCG5,anATP-binding cassette(ABC)transporter,isrequired for hypodermal suberization of rootsin rice(Oryza sativa).Plant J.80:40–51.Verrier,P.J.,et al.(2008).Plant ABC proteins—a unified nomenclature and updated inventory.Trends Plant Sci.13:151–159.Yadav,V.,Molina,I.,Ranathunge,K.,Castillo,I.Q.,Rothstein,S.J.,and Reed,J.W.(2014).ABCG transporters are required forsuberin and pollen wall extracellular bar-riers in Arabidopsis.Plant Cell26:3569–3588.Roots of the abcg2abcg6abcg20triple mutantare more permeable to dye.Increased stainingby propidium iodide(PI)in the stele of triplemutant roots compared with the wild type(Col).(Adapted from Yadav et al.[2014],Figure4E.)/cgi/doi/10.1105/tpc.114.132290The Plant Cell,Vol.26:3471,September2014,ã2014American Society of Plant Biologists.All rights reserved.DOI 10.1105/tpc.114.132290; originally published online September 23, 2014;2014;26;3471Plant Cell Nancy R. HofmannSupply Route: ABCG Transporters Act in the Construction of Suberin BarriersThis information is current as of February 8, 2015References/content/26/9/3471.full.html#ref-list-1This article cites 6 articles, 2 of which can be accessed free at:Permissionshttps:///ccc/openurl.do?sid=pd_hw1532298X&issn=1532298X&WT.mc_id=pd_hw1532298X eTOCs/cgi/alerts/ctmain Sign up for eTOCs at:CiteTrack Alerts/cgi/alerts/ctmain Sign up for CiteTrack Alerts at:Subscription Information/publications/subscriptions.cfm is available at:Plant Physiology and The Plant Cell Subscription Information for ADVANCING THE SCIENCE OF PLANT BIOLOGY© American Society of Plant Biologists。
细胞工程
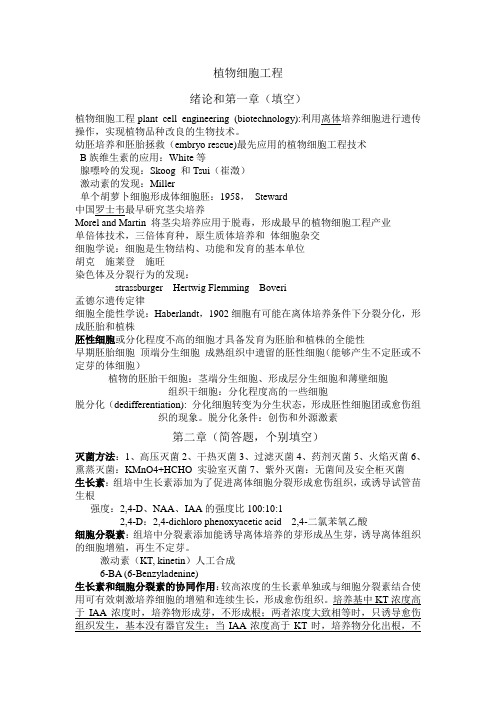
植物细胞工程绪论和第一章(填空)植物细胞工程plant cell engineering (biotechnology):利用离体培养细胞进行遗传操作,实现植物品种改良的生物技术。
幼胚培养和胚胎拯救(embryo rescue)最先应用的植物细胞工程技术B族维生素的应用:White等腺嘌呤的发现:Skoog 和Tsui(崔澂)激动素的发现:Miller单个胡萝卜细胞形成体细胞胚:1958,Steward中国罗士韦最早研究茎尖培养Morel and Martin 将茎尖培养应用于脱毒,形成最早的植物细胞工程产业单倍体技术,三倍体育种,原生质体培养和体细胞杂交细胞学说:细胞是生物结构、功能和发育的基本单位胡克施莱登施旺染色体及分裂行为的发现:strassburger Hertwig Flemming Boveri孟德尔遗传定律细胞全能性学说:Haberlandt,1902细胞有可能在离体培养条件下分裂分化,形成胚胎和植株胚性细胞或分化程度不高的细胞才具备发育为胚胎和植株的全能性早期胚胎细胞顶端分生细胞成熟组织中遗留的胚性细胞(能够产生不定胚或不定芽的体细胞)植物的胚胎干细胞:茎端分生细胞、形成层分生细胞和薄壁细胞组织干细胞:分化程度高的一些细胞脱分化(dedifferentiation): 分化细胞转变为分生状态,形成胚性细胞团或愈伤组织的现象。
脱分化条件:创伤和外源激素第二章(简答题,个别填空)灭菌方法:1、高压灭菌2、干热灭菌3、过滤灭菌4、药剂灭菌5、火焰灭菌6、熏蒸灭菌:KMnO4+HCHO 实验室灭菌7、紫外灭菌:无菌间及安全柜灭菌生长素:组培中生长素添加为了促进离体细胞分裂形成愈伤组织,或诱导试管苗生根强度:2,4-D、NAA、IAA的强度比100:10:12,4-D:2,4-dichloro phenoxyacetic acid 2,4-二氯苯氧乙酸细胞分裂素:组培中分裂素添加能诱导离体培养的芽形成丛生芽,诱导离体组织的细胞增殖,再生不定芽。
植物相关期刊
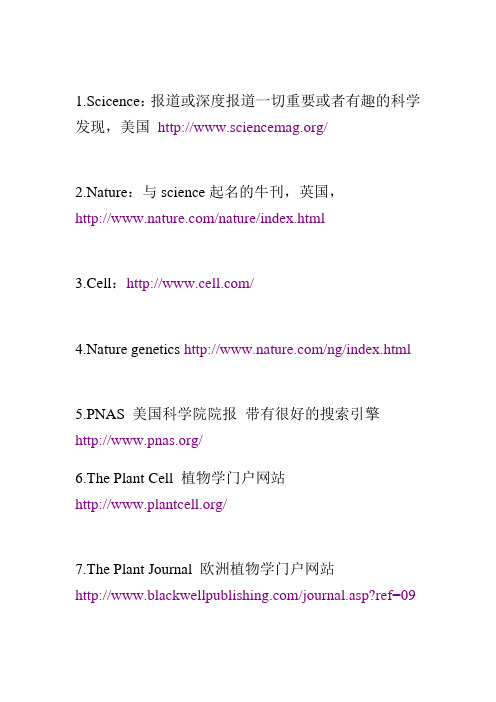
1.Scicence:报道或深度报道一切重要或者有趣的科学发现,美国/2.Nature:与science起名的牛刊,英国,/nature/index.html3.Cell:/4.Nature genetics /ng/index.html5.PNAS 美国科学院院报带有很好的搜索引擎/6.The Plant Cell 植物学门户网站/7.The Plant Journal 欧洲植物学门户网站/journal.asp?ref=0960-74128.Genetics遗传学门户/journal.asp?ref =0960-74129.Genome 基因组学门户http://pubs.nrc-cnrc.gc.ca/rp-ps/journalDetail.jsp?jcode= gen&lang=eng10.Genome research 基因组学新贵/11.Functional & Integrative Genomics 功能与系统基因组学/content/105695/12.Plant Cell Reports/content/100383/13.PMB 植物分子生物学门户/content/100330/14.Journal of Plant Res/content/108924/15.Mol Biol Rep 应用生物学研究/content/100316/16.TAG 理论与应用遗传学德国目前中国有4位牛人担任该期刊编委/new+%26+forthcoming+titles+ %28default%29/journal/12217.MGG 分子遗传与基因组学/content/107567/发表于 2008-11-23 02:26 | 只看该作者本帖最后由 sweetoath 于 2009-10-26 15:58 编辑18.Mol Cell 分子细胞/molecular-cell/home19.Molecular Breeding 分子育种/content/1572-9788/20.Crop Science 美国的《作物学报》/21.Plos 最近很牛的系列期刊/journals/index.php22.Plant Physiology 植物生理门户/23.Transgenic Research 转基因研究/content/100225/24.Methods in Molecular Biology分子生物学方法/series/765125.Trends in plant science了解牛人综述,把握植物科学研究进展的捷径/claim_online_access.htm26.CURR OPIN PLANT BIOL 同上/wps/find/journal_browse.cws_h ome27.中国科学/科学通报中国期刊门户/28.植物学报/new/cn/index.asp29.中国农业科学/v2/index.asp30.遗传学报国内遗传学情况了解渠道/31.作物学报中国的《作物科学》http://211.155.251.148:8080/zwxb/CN/volumn/current.s html32. JEB Journal of Experimental Botany,/33.Euphytica /life+sci /plant+sciences/journal/1068134. Journal of Cereal Science/wps/find/journaldescri ption.cws_home/622859/description#description35 Field crop research 大田作物研究36 Annual Review of Plant Science 超牛的综述合集,一年只发一期!!!http://arjournals.annualreviews. ...008&cookieSet=11评分次数。
The maize Lc regulatory gene up-regulates the flavonoid
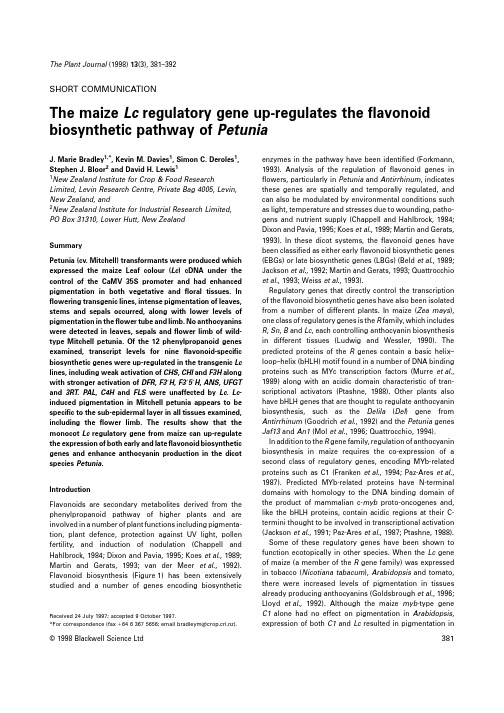
The Plant Journal (1998)13(3),381–392SHORT COMMUNICATIONThe maize Lc regulatory gene up-regulates the flavonoid biosynthetic pathway of PetuniaJ.Marie Bradley 1,*,Kevin M.Davies 1,Simon C.Deroles 1,Stephen J.Bloor 2and David H.Lewis 11New Zealand Institute for Crop &Food ResearchLimited,Levin Research Centre,Private Bag 4005,Levin,New Zealand,and2New Zealand Institute for Industrial Research Limited,PO Box 31310,Lower Hutt,New Zealand SummaryPetunia (cv.Mitchell)transformants were produced which expressed the maize Leaf colour (Lc )cDNA under the control of the CaMV 35S promoter and had enhanced pigmentation in both vegetative and floral tissues.In flowering transgenic lines,intense pigmentation of leaves,stems and sepals occurred,along with lower levels of pigmentation in the flower tube and limb.No anthocyanins were detected in leaves,sepals and flower limb of wild-type Mitchell petunia.Of the 12phenylpropanoid genes examined,transcript levels for nine flavonoid-specific biosynthetic genes were up-regulated in the transgenic Lc lines,including weak activation of CHS ,CHI and F3H along with stronger activation of DFR ,F3ЈH ,F3Ј5ЈH ,ANS ,UFGT and 3RT .PAL ,C4H and FLS were unaffected by Lc .Lc -induced pigmentation in Mitchell petunia appears to be specific to the sub-epidermal layer in all tissues examined,including the flower limb.The results show that the monocot Lc regulatory gene from maize can up-regulate the expression of both early and late flavonoid biosynthetic genes and enhance anthocyanin production in the dicot species Petunia .IntroductionFlavonoids are secondary metabolites derived from the phenylpropanoid pathway of higher plants and are involved in a number of plant functions including pigmenta-tion,plant defence,protection against UV light,pollen fertility,and induction of nodulation (Chappell and Hahlbrock,1984;Dixon and Pavia,1995;Koes et al .,1989;Martin and Gerats,1993;van der Meer et al .,1992).Flavonoid biosynthesis (Figure 1)has been extensively studied and a number of genes encoding biosyntheticReceived 24July 1997;accepted 9October 1997.*For correspondence (fax ϩ6463675656;email bradleym@).©1998Blackwell Science Ltd381enzymes in the pathway have been identified (Forkmann,1993).Analysis of the regulation of flavonoid genes in flowers,particularly in Petunia and Antirrhinum ,indicates these genes are spatially and temporally regulated,and can also be modulated by environmental conditions such as light,temperature and stresses due to wounding,patho-gens and nutrient supply (Chappell and Hahlbrock,1984;Dixon and Pavia,1995;Koes et al .,1989;Martin and Gerats,1993).In these dicot systems,the flavonoid genes have been classified as either early flavonoid biosynthetic genes (EBGs)or late biosynthetic genes (LBGs)(Beld et al .,1989;Jackson et al .,1992;Martin and Gerats,1993;Quattrocchio et al .,1993;Weiss et al .,1993).Regulatory genes that directly control the transcription of the flavonoid biosynthetic genes have also been isolated from a number of different plants.In maize (Zea mays ),one class of regulatory genes is the R family,which includes R ,Sn ,B and Lc ,each controlling anthocyanin biosynthesis in different tissues (Ludwig and Wessler,1990).The predicted proteins of the R genes contain a basic helix–loop–helix (bHLH)motif found in a number of DNA binding proteins such as MYc transcription factors (Murre et al .,1989)along with an acidic domain characteristic of tran-scriptional activators (Ptashne,1988).Other plants also have bHLH genes that are thought to regulate anthocyanin biosynthesis,such as the Delila (Del )gene from Antirrhinum (Goodrich et al .,1992)and the Petunia genes Jaf13and An1(Mol et al .,1996;Quattrocchio,1994).In addition to the R gene family,regulation of anthocyanin biosynthesis in maize requires the co-expression of a second class of regulatory genes,encoding MYb-related proteins such as C1(Franken et al .,1994;Paz-Ares et al .,1987).Predicted MYb-related proteins have N-terminal domains with homology to the DNA binding domain of the product of mammalian c-myb proto-oncogenes and,like the bHLH proteins,contain acidic regions at their C-termini thought to be involved in transcriptional activation (Jackson et al .,1991;Paz-Ares et al .,1987;Ptashne,1988).Some of these regulatory genes have been shown to function ecotopically in other species.When the Lc gene of maize (a member of the R gene family)was expressed in tobacco (Nicotiana tabacum ),Arabidopsis and tomato,there were increased levels of pigmentation in tissues already producing anthocyanins (Goldsbrough et al .,1996;Lloyd et al .,1992).Although the maize myb -type gene C1alone had no effect on pigmentation in Arabidopsis ,expression of both C1and Lc resulted in pigmentation in382J.Marie Bradley etal.Figure 1.Flavonoid pathway.Abbreviations are as follows:PAL,phenylalanine ammonia lyase;C4H,cinnamate 4-hydroxylase;CHS,chalcone synthase;CHI,chalcone isomerase;F3H,flavanone 3-hydroxylase;DFR,dihydroflavonol 4-reductase;FLS,flavonol synthase;F3ЈH,flavonoid 3Ј-hydroxylase;F3Ј5ЈH,flavonoid 3Ј,5Ј-hydroxylase;ANS,anthocyanidin synthase;UFGT,UDP-glucose:flavonoid 3-O -glucosyltransferase;3RT,UDP-rhamnose:anthocyanidin-3-glucoside rhamnosyltransferase;3MT,anthocyanin methyltransferase.tissues that normally did not produce anthocyanins (Lloyd et al .,1992).Ectopic expression of the Del gene also led to increased anthocyanins in the solanaceous species tobacco and tomato (Mooney et al .,1995).However,Del appears to have a reduced range of action in terms of plant species because,in contrast to Lc ,Del had little or no effect on pigmentation in Arabidopsis (Mooney et al .,1995).Flavonoid biosynthetic gene expression in another solana-ceous species,Petunia,also appears to be affected by heterologous monocot regulatory genes.In transient expression assays in petunia,the maize Lc and C1genes together were shown to up-regulate the promoter of the dihydroflavonol 4-reductase (DFR )gene fused to the GUS reporter gene (Quattrocchio et al .,1993).However,bom-bardment with Lc alone had little or no effect on the DFR promoter–GUS construct or on pigmentation.A single petunia line expressing Lc was generated but as this did ©Blackwell Science Ltd,The Plant Journal ,(1998),13,381–392not survive into maturity no further analysis was presented (Quattrocchio et al .,1993).We have introduced the maize Lc cDNA,under the control of the cauliflower mosaic viral (CaMV)35S promoter,into the white-flowered Mitchell (W115)mutant of petunia and have generated a number of stably transformed lines.Mitchell carries mutated alleles of two regulatory loci (an2and an4).An2controls anthocyanin biosynthesis in the flower limb,and An4regulates pigmentation in the anther and,to a lesser degree,the tube (Quattrocchio et al .,1993;Wiering and de Vlaming,1984).These two loci have been shown to control expression of several genes in the fla-vonoid pathway including DFR ,UDP-glucose:flavonoid glycosyltransferases (UFGT )and methyltransferase (MT )(Beld et al .,1989;Huits et al .,1994;Quattrocchio et al .,1993).Mitchell was chosen because initial evidence sug-gested that both An2(Quattrocchio et al .,1993)and An4Lc up-regulatesflavonoid biosythesis in petunia383(Quattrocchio,1994)may have been bHLH factors.It is only recently that work has shown that An2(Quattrocchio, 1994)and possibly An4encode Myb-like transcription fac-tors(Mol et al.,1996).We show here that the expressionof the Lc cDNA,under the control of the CaMV35S promoter,results in anthocyanin production in the leaves along with increased pigmentation in stems,sepal,flower tube and limb of Mitchell.The presence of Lc leads to an increasedflux through theflavonoid pathway via the up-regulation of a number of the petuniaflavonoid biosyn-thetic genes.ResultsGeneration of transgenic petunia lines with enhanced pigmentationA2.2kb Lc cDNA,lacking the5Јuntranslated region that can limit translation(Damiani and Wessler,1993),was placed under the control of the CaMV35S promoter(see Experimental procedures)and introduced into Mitchell petunia via Agrobacterium-mediated transformation (Deroles and Gardner,1988).Twenty-two independent transformants were produced from160leaf pieces,repres-enting a transformation efficiency of13.7%.Although a number of the shoots able to proliferate on kanamycin showed a range of pigmentation in their leaves and stems, the new growth of all of the transgenic plantlets showed little or no coloration after transfer to the glasshouse. However,as the plants matured,nine of the22independent transgenic lines displayed a steady increase in the levelof pigmentation in the leaves.Leaves produced later in development,particularly after the transition toflowering, showed the greatest levels of pigment,resulting in foliage with purple/black coloration(an example is shown in Figure2b).Pigmentation not only increased during plant development but also under conditions of high light and stress(data not shown).Untransformed lines of Mitchell had no pigment in the leaves when grown in culture or under glasshouse conditions but did display a reddeningof the stem and the calyx of the inflorescences,particularlyin high light.The levels of pigmentation in these tissues were enhanced in the Lc transgenics expressing Lc (Figure2a,b).Under our glasshouse conditions,Mitchell generally pro-ducesflowers with entirely white limbs and low levels of pigmentation in the outer veins and the inner throat of theflower tube(Figure2c,d).The outsides of theflower tubesof the Lc transgenics were often more pigmented than the untransformedflowers(Figure2d),as was the outer marginof the limb of the Lc lines,where the pigment was often associated with the veins(Figure2c).Limb pigmentation was most intense in theflower buds prior to opening and declined significantly in the fully openflower.The©Blackwell Science Ltd,The Plant Journal,(1998),13,381–392pigmentation of the Lcflowers,like that of the leaves, appeared to be somewhat variable and may also have been influenced by factors such as high light levels.Pigmentation in the progeny of the Lc transgenic linesT2seedlings of the primary Lc transgenic lines displayed a reddening of cotyledons and hypocotyl(Figure2e). Enhanced pigmentation also occurred in newly developing true leaves but was no longer detectable when those early leaves became fully expanded.The T2progeny of the most highly pigmented line(38–110)were again highly pigmented but grew very slowly,often not developing beyond the cotyledon stage(data not shown).The progeny that did reach maturity showed a marked reduction in pigmentation,which was present only in sectors of the leaves.Light-grown roots of T2progeny from selfed trans-formants or plants back-crossed to Mitchell were not pig-mented.However,when the Lc transformants were out-crossed to a coloured petunia line(V26),T2progeny with the Lc-like phenotype in the cotyledons were also pig-mented in the main root when grown in the light (Figure2e).A consistent effect on pigmentation,particularly of the vegetative tissues,was seen in a number of different petunia backgrounds that contained Lc(data not shown). The kanamycin-resistant T3progeny of crosses between the Mitchell Lc transgenics and V26,or two commercially available petunia cultivars,all showed enhanced levels of pigmentation in the leaves,stems and sepals.Due to a high level of variability inflower colour in the T3progeny from both untransformed control and Lc crosses,it was difficult to assess whether Lc had affected pigmentation in thefloral tissues of these other petunia lines.Spatial location of pigmentThe spatial location of the pigment within tissues of both untransformed Mitchell controls and the Lc transgenics was examined.In the Lc lines,the pigment was mainly in the palisade cell layer of the leaves rather than the epi-dermis(Figure3a,b)and the level of pigmentation varied considerably between individual palisade cells(Figure3b). Although the degree of pigmentation of the underside of the Lc leaves varied during development,the pigment was also located sub-epidermally,in the mesophyll cells (Figure3a).No pigmentation was observed in cells of Mitchell leaves.In the pigmented stems of both Mitchell and the Lc transgenic lines,the pigment was sub-epidermal (Figure3c,d).Pigmentation in untransformed Mitchell sepals was also sub-epidermal and was associated with the main sepal vein(Figure3e).In contrast,in Lc plants, the sepals(Figure3f)and the connective tissue between the sepals were pigmented throughout the sub-epidermal384J.Marie Bradley et al.©Blackwell Science Ltd,The Plant Journal,(1998),13,381–392Lc up-regulates flavonoid biosythesis in petunia385layer.Pigmentation in the vegetative tissues such as the petiole of the leaf,the stem and sepals of V26was also sub-epidermal (data not shown).In Mitchell flower tubes,pigmentation is sub-epidermal and located near to the vascular bundles running along the tube.Pigmentation in the Lc transgenics remained sub-epidermal and often associated with the vascular tissue;however,a further mosaic of sub-epidermal cells between the veins was pigmented (data not shown).The location of pigment in the limb of the flowers of Lc plants was particularly interesting.Although anthocyanins in coloured petunia lines,such as V26,are located in the epidermis of the limb,particularly on the inner or front side of the petal (Figure 3g),the pigment in the limb of Lc flowers occurred in the sub-epidermal layer of the outer (or rear)face of the limb (Figure 3h).Leaf pigmentation is related to the presence of the Lc transcriptAlthough the Lc transgene was detected in 12of the 13transgenic lines tested,Lc expression was only detected in those lines displaying an altered phenotype (data not shown).Transformant 38–110had the highest levels of the Lc transcript and the most highly pigmented phenotype,whereas 38–118had a lower expression of the Lc transgene and a more intermediate level of pigmentation.Southern analysis and kanamycin inheritance suggested that 38–110carried multiple T-DNA inserts and 38–118contained a single,heritable T-DNA locus (data not shown).The pig-mented phenotype of 38–118co-segregated with kanamy-cin resistance and the presence of the Lc cDNA and transcript (Figure 4).Southern analysis suggested that the T 2progeny 475and 465were heterozygous and 472and 467were homozygous for the Lc transgene (Figure 4b),which was confirmed by the kanamycin resistance ratios in the T 3progeny (data not shown).The levels of Lc transcript were higher in the homozygous T 2plants com-pared with the heterozygous lines (Figure 4c).The degree of pigmentation correlated with the level of Lc expression in both the primary transformants (data not shown)and the T 2progeny (Figure 4e).Figure 2.Transgenic Lc petunia phenotypes.Phenotype of homozygous T 2progeny of Lc transgenic line 38–118,carrying two (line 472)or no copies (line 480)of the Lc transgene T-DNA construct.Refer to text and Figure 4for additional details on individual progeny.(a)Shoot,just prior to plant bolting to flowering,line 480(control).(b)Shoot of line 472(Lc ).Early-stage flower bud development can be seen.(c)Flower limb of lines 480(upper)and 472(lower).(d)Flower tube of lines 480(upper)and 472(lower).Upper regions of sepals can also be seen.(e)T 2seedlings of 38–118ϫV26grown under high light.Non-pigmented,untransformed seedling (left)and Lc seedling with pigmented cotyledons,upper hypocotyl and root (right).©Blackwell Science Ltd,The Plant Journal ,(1998),13,381–392Flavonoid profile is altered in the Lc transgenicsA detailed quantitative analysis of untransformed Mitchell control and the two primary Lc transformants,38–110and 38–118,showed that the levels of flavonoids were altered in several tissues of the transgenics (Figure 5a,b).No anthocyanins were detected in the leaves,sepals or limb of the Mitchell control.The transgenics contained anthocy-anins in all these tissues,with the highest concentrations detected in the leaves (Figure 5a).Although the tube in Mitchell flowers does contain some anthocyanins,the levels were also increased in the Lc transgenics.Unlike the anthocyanins,flavonols were detected in all the Mitchell tissues examined (Figure 5b).The flavonol levels in the sepal and tube of both transgenic lines were higher than the levels in Mitchell.However,little,if any,increase in the flavonol levels was observed in the limb of the Lc transformants.The leaves of 38–118appeared to have reduced flavonol levels compared with the Mitchell control.Lc affects flavonoid biosynthetic gene expression Figure 6shows a detailed analysis of flavonoid biosynthetic gene expression in various tissues of two Lc transgenic lines,38–110and 38–118,and an untransformed Mitchell control.Lc expression was found in leaf,sepals,tube and limb of both transgenics (Figure 6).Similar to previous reports (Benfey and Chua,1989,1990),expression from the CaMV 35S promoter in petunia appeared to be highest in the limb,lower in the tube and lower still in sepal and leaves,with the exception of a high level of expression in the leaves of 38–110.Lc expression in the 38–118leaf sample shown appeared to be abnormally low due to reduced RNA loading in this lane (Figure 6).The expression of Lc in petunia led to an increase in transcript levels of many of the flavonoid biosynthetic genes.The highest degree of up-regulation was seen with the flavonoid biosynthetic genes DFR ,flavonoid 3Ј5Јhydroxylase (F3Ј5ЈH ),anthocyanidin synthase (ANS ),UFGT and rhamnosyltransferase (3RT )(Figure 6).In Mitchell,tran-script levels for these genes were close to,or below,detectable levels in the leaves and sepals,and at slightly higher levels in the flower tube and limb (with the exception386J.Marie Bradley et al.©Blackwell Science Ltd,The Plant Journal,(1998),13,381–392Lc up-regulates flavonoid biosythesis in petunia387of DFR and F3Ј5ЈH which were not detectable in limbs).However,in the Lc transgenic lines,there was a clear increase in transcript levels for DFR ,F3Ј5ЈH ,ANS ,UFGT and 3RT in all four tissues (Figure 6).In untransformed Mitchell,the EBGs chalcone synthase (CHS ),chalcone isomerase (CHI )and flavanone 3-hydroxylase (F3H )are expressed in all four tissues,at relatively low levels in leaves,sepal and tube and at high levels in the limb of the flower (Figure 6).Expression of these genes was also increased by Lc as seen by the small but clear increase in transcript levels,particularly in the leaf,sepal and tube of the transgenics (Figure 6).The increased expression was less dramatic in the limbs because of the already high level of expression in Mitchell control tissue but a slight increase was visible using with shorter exposures of the autoradiographs (data not shown).The flavonoid 3Ј-hydroxylase gene (F3ЈH )appeared to be affected differently by Lc compared with the other up-regulated flavonoid biosynthetic genes.Control Mitchell leaves and sepals had low levels of F3ЈH transcript,with higher expression in the tube and limb of the flower (Figure 6).In both Lc transgenic lines,there appeared to be a slight increase in F3ЈH expression in tube and limb,but,in contrast to the other genes,little or no induction in F3ЈH expression in leaves and sepals.A similar induction of flavonoid gene expression was seen in subsequent experiments with T 2progeny of 38–118.For instance,in leaf samples of the segregating T 2progeny (shown in Figure 4),enhanced gene expression was only seen in those plants expressing Lc (data not shown).In addition,analysis of heterozygous T 2progeny (from a backcross of 38–118to Mitchell),those lines expressing Lc showed the same weak activation of EBGs and stronger activation of LBGs in the leaves from young,vegetative and more mature,flowering plants along with enhanced expression in sepals,tubes and limbs of the flowers compared with non-expressing lines (data not shown).There were some genes in the pathway that were unaf-fected by Lc .No effect was seen on phenylalanine ammonia lyase (PAL )and cinnamate 4-hydroxylase (C4H )expression in either leaf,sepal or flower tissue (Figure 6).Differences in the age of tissue,particularly the leaf samples,mayFigure 3.Spatial location of pigmentation.Cross-sections through homozygous T 2progeny of Lc transgenic line 38–118,carrying two (line 472)or no copies (line 480)of the Lc transgene T-DNA construct.Refer to text and Figure 4.for additional details on individual progeny.The scale bars for (a)–(d)represents 0.1mm actual size and those for (e)–(h)represent 0.05mm.(a),(b)Leaf tissue of line 472(Lc ).(c)Stem tissue of line 480(control).(d)Stem tissue of line 472(Lc ).(e)Sepal tissue of line 480(control).(f)Sepal tissue of line 472(Lc ).(g)Flower limb of purple-flowered Mitchell ϫV26F 2plant.(h)Flower limb of line 472(Lc ).©Blackwell Science Ltd,The Plant Journal ,(1998),13,381–392explain the slight variation in PAL and C4H expression between the lines in Figure 6,as both PAL and C4H are developmentally regulated during petunia leaf develop-ment (unpublished data).Flavonols are produced by fla-vonol synthase (FLS)as a side branch en route to anthocyanin production (see Figure 1).FLS was most highly expressed in the limbs,followed by the tube,leaf and sepal of untransformed Mitchell.Lc had no discernible effects on FLS transcript levels,as can be seen in the Lc transgenics (Figure 6).DiscussionWe present here a detailed analysis of the effect of the monocot Lc regulatory gene from maize on flavonoid biosynthesis in a dicotyledonous system,ing plants that stably expressed Lc and that had enhanced levels of pigmentation,we examined changes in general flavonoid biosynthesis and in the expression of 12genes of the phenylpropanoid pathway.We also determined the amount and localization of the anthocyanins induced.We were able to produce viable,fertile,transgenic Mitchell petunia lines containing the Lc cDNA under the control of the highly expressed 35S CaMV promoter.Expression of Lc greatly enhanced pigmentation in leaves,stems,sepals and flower tube and limb of the transformants (Figure 2).However,in some situations,the expression of Lc may have a detrimental effect on plant growth.The transformation efficiency when using the Lc cDNA con-struct was only 13.7%,compared with an average rate of 23.5%obtained in similar experiments with four other vectors that contained different cDNAs but used the same base binary (unpublished data).Also,the pigmented T 2seedlings of the highest Lc expressing line (38–110)were severely stunted and did not develop beyond the cotyledon stage (data not shown).Similar growth inhibition was observed in both tomato and Arabidopsis lines transformed with Lc (Goldsbrough et al .,1996;Lloyd et al .,1994).Further,in previous attempts to stably express Lc in petu-nia,only a single,aberrant transformant was produced,as either the pigmented calli failed to give rise to shoots,or the shoots failed to root (Quattrocchio et al .,1993).This suggests that there is some threshold level at which either388J.Marie Bradley etal.Figure 4.Analysis of the T 2progeny of Lc transgenic 38–118.The T 2progeny of a selfed cross of 38–118were examined in detail.(a)Presence of the Lc transgene in T 2progeny.Southern blot of approximately 5µg of genomic DNA digested with Eco RV which,by cutting within the 35S promoter and pTi5Ј7Јterminator,produces an internal T-DNA fragment of approximately 2.3kb that contains the Lc cDNA.The membrane was probed with radioactively labelled Lc349Xba I insert,and DNA loadings were shown to be approximately equal through hybridization to the endogenous petunia ANS gene probe pCGP1407(data not shown).(b)Lc expression in T 2progeny.Each lane contains 10µg of total RNA from expanding leaf tissue and was probed with radioactively labelled Lc349Xba I insert.(d)Kanamycin resistance of leaf discs.Leaf discs were sterilized and placed on medium containing 200p.p.m.kanamycin.Leaf discs able to regenerate callus followed by shoot development were judged to be kanamycin-resistant (KR).Kanamycin-sensitive discs (KS)did not produce callus and eventually yellowed in response to the kanamycin.(e)Anthocyanin levels (mg g –1dry weight)in the leaves of plants during vegetative phase of growth.the Lc protein itself becomes toxic or at which it may be regulating another pathway inhibitory to growth.Alternat-ively,very high levels of anthocyanins may be detrimental to the plant.Mitchell petunia carries mutant alleles of the regulatory loci an2and an4,both of which control flavonoid biosyn-thesis in the flower.Neither loci were functionally comple-mented by the Lc product.Given that recent studies show that An2and perhaps An4encode Myb-type factors (Quattrocchio,1994;Mol et al .,1996),complementation by the bHLH Lc factor would no longer be expected.The original transient expression studies that suggested that Lc could complement the mutations in Mitchell flowers (Quattrocchio et al .,1993)probably reflect enhancement of pigmentation in sub-epidermal layers of the petal.The total level of anthocyanins in the sepal,tube,limb,and,in particular,the leaves of the transgenic Lc lines was significantly higher than in control plants (Figure 5).The degree of increased pigmentation and up-regulation of gene expression correlated to the level of Lc transcript (Figures 4and 6),suggesting a direct action of the Lc transgene.The major anthocyanins present in the tissues of the Lc transgenics were made up of acylated derivatives of the 3Ј5Јhydroxylated anthocyanin,petunidin-3-rutino-side-5-glucoside (S.Bloor,personal communication).These©Blackwell Science Ltd,The Plant Journal ,(1998),13,381–392Figure 5.Flavonoid concentration in Mitchell and Lc transgenics.(a)Anthocyanin concentration in mg g –1dry weight.(b)Flavonol concentration in mg g –1dry weight (rutin equivalents).Three measurements of each extract were made.Stage 4,almost fully opened flowers,were used for the sepal,tube and limb samples.Leaf tissue of 38–110was not measured for flavonoid concentration.results suggest that Lc is activating a similar set of flavonoid genes in the different petunia tissues.In a comprehensive analysis of the effect of Lc on the expression of the petunia flavonoid biosynthetic genes,we found that Lc strongly up-regulates the LBGs such as DFR ,F3ЈH ,F3Ј5ЈH ,ANS ,UFGT and 3RT,and,more weakly,the EBGs including CHS ,CHI and F3H (Figure 6).In maize,R genes such as Lc ,together with C1,can regulate both EBGs and LBGs including CHS ,F3H ,DFR,UFGT and Bz2(Deboo et al .,1995;Dooner,1983;Dooner and Nelson,1979;Ludwig et al .,1989;Reddy et al .,1987).The results presented here extend previous findings in which Lc and Del were shown to up-regulate DFR and,to a lesser extent,CHS in tobacco and also,in the case of Del ,in tomato (Mooney et al .,1995).We were also able to show,for the first time,an effect of a flavonoid regulatory gene on the expression of F3ЈH and F3Ј5ЈH genes.Like the LBGs examined (DFR ,ANS ,UFGT and 3RT ),F3Ј5ЈH expression was up-regulated by Lc in most petunia tissues (Figure 6).However,in a surprising contrast to the other affected flavonoid genes,Lc appar-ently had a differential effect on F3ЈH expression,with littleLc up-regulatesflavonoid biosythesis in petunia389©Blackwell Science Ltd,The Plant Journal,(1998),13,381–392or no induction in leaves and sepals but some up-regulation of F3ЈH transcript levels inflower tube and limb(Figure6). In petunia,many of theflavonoid genes such as CHS,CHI, DFR,F3ЈH,F3Ј5ЈH,UFGT and methylases are known to belong to multi-gene families(Beld et al.,1989;Jonsson et al.,1983;Koes et al.,1989;van Tunen et al.,1988;Wiering and de Vlaming,1984).In some cases,individual family members have been shown to have distinct expression patterns and/or are controlled by different environmental or developmental signals(Koes et al.,1989;van Tunen et al.,1988).The Lc up-regulation of most of the petunia genes occurred in all the tissues examined,including leaves,sepals,flower tube and limb,which suggests that Lc is capable of up-regulating at least one or more of these members in different tissues.There are at least two loci, Ht1and Ht2,that encode F3ЈH(Wiering and de Vlaming, 1984),so the differential regulation of F3ЈH by Lc could be due to the up-regulation of a particular F3ЈH gene member that is expressed infloral tissues but not in vegetative ones.We have measured total transcript levels encoding the biosynthetic enzymes and further research would be required to determine which individual family members are activated by Lc.Although Lc is able to activate both EBGs and LBGs in petunia,its effect appears to be specific to genes required for anthocyanin biosynthesis as there was no change in the expression of genes such as PAL,C4H and FLS (Figure6).PAL and C4H which act early in the phenylpro-panoid pathway,are required for the synthesis offlavo-noids,lignins and stilbenes and are not solely committed to anthocyanin biosynthesis(see Figure1).FLS synthesizes flavonols as a side branch en route to anthocyanin produc-tion,competing with DFR for the dihydroflavonol sub-strates(Figure1).Little is known about the regulation of the biosynthesis offlavonols but our results suggest that it is under separate control to that of the anthocyanins,as Lc had no discernible effect on the regulation of FLS expression in any of the tissues examined(Figure6).The small enhancement offlavonol levels observed in the Lc transgenics,particularly in sepal and tube,may be due to increasedflux through the mainflavonoid pathway, providing more substrate for conversion intoflavonols. Lc and Del,when expressed in dicots,appear to be up-regulating existingflavonoid pathways that are already expressed at low levels.In Mitchell petunia,the stem, sepals,flower tube and occasionally leaves andflower limb show some pigmentation in conditions such as highFigure6.Gene expression in Mitchell and Lc transgenics.Replicate membranes with10µg of total RNA from leaf(a mixture of developmental stages),sepal,tube and limb tissue of a mix of stage2–4flowers from Mitchell(MP)and primary Lc transgenic lines38–110and38–118were hybridized to radioactively labelled probes as described in Experimental procedures.。
SCI收录的植物学类期刊介绍_王彦

4. 296 4. 257 4. 149 4. 085 4. 01 3. 821 3. 709 3. 388 3. 277 3. 264 3. 15 3. 098 3. 067 3. 052 2. 872 2. 792 2. 773
中国 日本 荷兰 英国 美国 美国 英国 英国 英国 德国 英国 德国 丹麦 美国 美国 捷克 荷兰
AND SYSTEMATICS 14 MOLECULAR PLANT 15 PLANT AND CELL PHYSIOLOGY 16 PLANT MOLECULAR BIOLOGY 17 BMC PLANT BIOLOGY 18 MOLECULAR PLANT-MICROBE INTERACTIONS 19 CRITICAL REVIEWS IN PLANT SCIENCES 20 MOLECULAR PLANT PATHOLOGY 21 ANNALS OF BOTANY 22 PLANT METHODS 23 THEORETICAL AND APPLIED GENETICS 24 PHYTOCHEMISTRY 25 PLANTA 26 PHYSIOLOGIA PLANTARUM 27 AMERICAN JOURNAL OF BOTANY 28 JOURNAL OF NATURAL PRODUCTS 29 PRESLIA 30 PLANT AND SOIL
2 期刊调查结果
JCR 是 ISI 出版的网络版期刊引用报告,它对包括 SCI 收录的 3 783 种期刊在内的 8 355 种期刊之 间的引用和被引用数据进行统计、运算,并针对每种期刊定义了影响因子等指数加以报道。JCR 是
* 基金项目: 中国科学院知识创新工程重要方向项目 ( KZCX2-YW-307) 收稿日期: 2011-09-14,2011-11-09 接受发表 作者简介: 王彦 ( 1957-) 女,副研究员,主要从事信息资源开发与科技文献信息检索工作。E-mail: Wangyan@ ms. xjb. ac. cn
the plant cell 模板 -回复
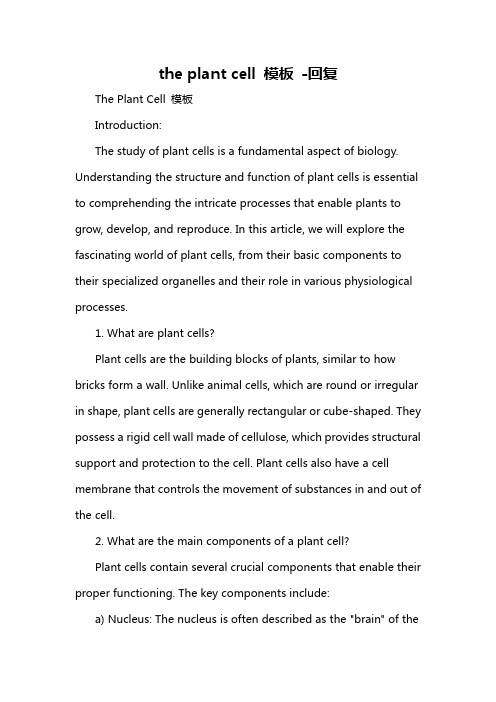
the plant cell 模板-回复The Plant Cell 模板Introduction:The study of plant cells is a fundamental aspect of biology. Understanding the structure and function of plant cells is essential to comprehending the intricate processes that enable plants to grow, develop, and reproduce. In this article, we will explore the fascinating world of plant cells, from their basic components to their specialized organelles and their role in various physiological processes.1. What are plant cells?Plant cells are the building blocks of plants, similar to how bricks form a wall. Unlike animal cells, which are round or irregular in shape, plant cells are generally rectangular or cube-shaped. They possess a rigid cell wall made of cellulose, which provides structural support and protection to the cell. Plant cells also have a cell membrane that controls the movement of substances in and out of the cell.2. What are the main components of a plant cell?Plant cells contain several crucial components that enable their proper functioning. The key components include:a) Nucleus: The nucleus is often described as the "brain" of thecell. It contains DNA, the genetic code that directs the synthesis of proteins and controls the cell's activities.b) Cytoplasm: The cytoplasm is a gel-like substance that fills the space between the cell membrane and the nucleus. It houses various organelles and serves as a medium for metabolic reactions.c) Endoplasmic reticulum (ER): The ER is a network of interconnected tubes and sacs that help transport proteins and lipids throughout the cell.d) Golgi apparatus: The Golgi apparatus is responsible for modifying, sorting, and packaging proteins and lipids into vesicles for transport within or outside the cell.e) Mitochondria: Often referred to as the "powerhouse" of the cell, mitochondria produce energy in the form of ATP through cellular respiration.f) Plastids: Plastids are specialized organelles found only in plant cells. Chloroplasts, a subtype of plastid, are responsible for photosynthesis, which converts sunlight into chemical energy.g) Vacuoles: Vacuoles are large, fluid-filled sacs that store water, nutrients, and waste materials. They also help maintain turgor pressure, which is important for plant rigidity.3. How do plant cells reproduce?Plant cells reproduce through a process called cell division.There are two types of cell division in plants: mitosis and meiosis.Mitosis occurs in somatic cells and serves to produce two identical daughter cells. This process is essential for growth, tissue repair, and asexual reproduction in plants. During mitosis, the DNA replicates, and the nucleus divides, resulting in the formation of two genetically identical daughter cells.Meiosis, on the other hand, occurs in specialized reproductive cells, called gametes, and leads to the formation of genetically diverse offspring. Meiosis involves two consecutive divisions, resulting in the production of four non-identical haploid cells.4. What are the unique features of plant cells?Some unique features of plant cells include:a) Cell wall: Plant cells have a rigid cell wall outside the cell membrane, providing strength and support to the cell.b) Chloroplasts: The presence of chloroplasts enables plants to carry out photosynthesis and convert sunlight into energy-rich molecules.c) Large central vacuole: Plant cells have a single, large central vacuole that helps maintain turgor pressure and store essential substances like water, ions, and metabolites.d) Plasmodesmata: Plasmodesmata are minute channels that connect adjacent plant cells, allowing the exchange of materialsand communication between cells.Conclusion:Plant cells are extraordinary entities that play vital roles in the growth and development of plants. From their unique structures and organelles to their role in reproduction and physiological processes, plant cells are essential components of the natural world. By further exploring and understanding these intricate entities, we can unlock the secrets of plant life and harness their potential for various applications.。
endnote plant cell copy -回复
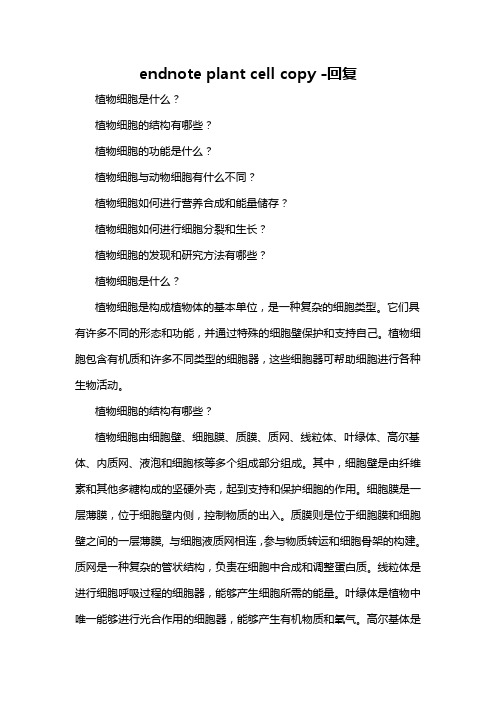
endnote plant cell copy -回复植物细胞是什么?植物细胞的结构有哪些?植物细胞的功能是什么?植物细胞与动物细胞有什么不同?植物细胞如何进行营养合成和能量储存?植物细胞如何进行细胞分裂和生长?植物细胞的发现和研究方法有哪些?植物细胞是什么?植物细胞是构成植物体的基本单位,是一种复杂的细胞类型。
它们具有许多不同的形态和功能,并通过特殊的细胞壁保护和支持自己。
植物细胞包含有机质和许多不同类型的细胞器,这些细胞器可帮助细胞进行各种生物活动。
植物细胞的结构有哪些?植物细胞由细胞壁、细胞膜、质膜、质网、线粒体、叶绿体、高尔基体、内质网、液泡和细胞核等多个组成部分组成。
其中,细胞壁是由纤维素和其他多糖构成的坚硬外壳,起到支持和保护细胞的作用。
细胞膜是一层薄膜,位于细胞壁内侧,控制物质的出入。
质膜则是位于细胞膜和细胞壁之间的一层薄膜, 与细胞液质网相连,参与物质转运和细胞骨架的构建。
质网是一种复杂的管状结构,负责在细胞中合成和调整蛋白质。
线粒体是进行细胞呼吸过程的细胞器,能够产生细胞所需的能量。
叶绿体是植物中唯一能够进行光合作用的细胞器,能够产生有机物质和氧气。
高尔基体是负责合成和分泌蛋白质的细胞器。
内质网则负责蛋白质的合成和运输。
液泡是含有细胞液和溶质的膜包裹结构,参与物质储存和细胞保护。
细胞核是细胞的控制中心,负责维持细胞的基因组和基因表达。
植物细胞的功能是什么?植物细胞的功能可以分为三个主要方面:营养合成和能量储存、细胞分裂和生长、以及分化和特殊化。
首先,植物细胞通过光合作用合成有机物质,其中叶绿体在光合作用中起到关键作用。
光合作用利用太阳能将二氧化碳和水转化为葡萄糖和氧气。
植物细胞通过合成和贮藏蛋白质和淀粉等物质来储存能量,并通过线粒体中进行呼吸作用来释放能量。
其次,植物细胞通过细胞分裂和生长实现体积的增加。
细胞分裂是细胞生命周期中的重要过程,通过有丝分裂和无丝分裂两种方式进行。
细胞生长涉及生物大分子的合成和细胞物质的积累,从而导致细胞体积的增加。
植物细胞工程Plant Cell Engineering
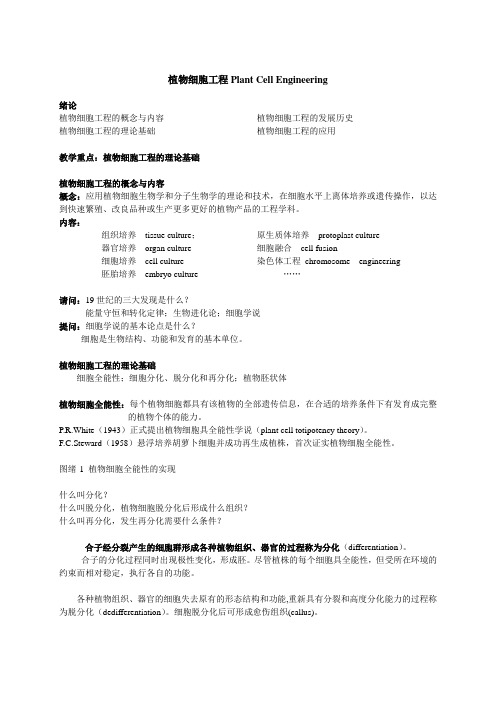
植物细胞工程Plant Cell Engineering 绪论植物细胞工程的概念与内容植物细胞工程的理论基础植物细胞工程的发展历史植物细胞工程的应用教学重点:植物细胞工程的理论基础植物细胞工程的概念与内容概念:应用植物细胞生物学和分子生物学的理论和技术,在细胞水平上离体培养或遗传操作,以达到快速繁殖、改良品种或生产更多更好的植物产品的工程学科。
内容:组织培养tissue culture;器官培养organ culture 细胞培养cell culture胚胎培养embryo culture 原生质体培养protoplast culture细胞融合cell fusion染色体工程chromosome engineering ……请问:19世纪的三大发现是什么?能量守恒和转化定律;生物进化论;细胞学说提问:细胞学说的基本论点是什么?细胞是生物结构、功能和发育的基本单位。
植物细胞工程的理论基础细胞全能性;细胞分化、脱分化和再分化;植物胚状体植物细胞全能性:每个植物细胞都具有该植物的全部遗传信息,在合适的培养条件下有发育成完整的植物个体的能力。
P.R.White(1943)正式提出植物细胞具全能性学说(plant cell totipotency theory)。
F.C.Steward(1958)悬浮培养胡萝卜细胞并成功再生成植株,首次证实植物细胞全能性。
图绪-1 植物细胞全能性的实现什么叫分化?什么叫脱分化,植物细胞脱分化后形成什么组织?什么叫再分化,发生再分化需要什么条件?合子经分裂产生的细胞群形成各种植物组织、器官的过程称为分化(differentiation)。
合子的分化过程同时出现极性变化,形成胚。
尽管植株的每个细胞具全能性,但受所在环境的约束而相对稳定,执行各自的功能。
各种植物组织、器官的细胞失去原有的形态结构和功能,重新具有分裂和高度分化能力的过程称为脱分化(dedifferentiation)。
PlantCell南方科大郭红卫教授团队发现植物衰老调控新机制
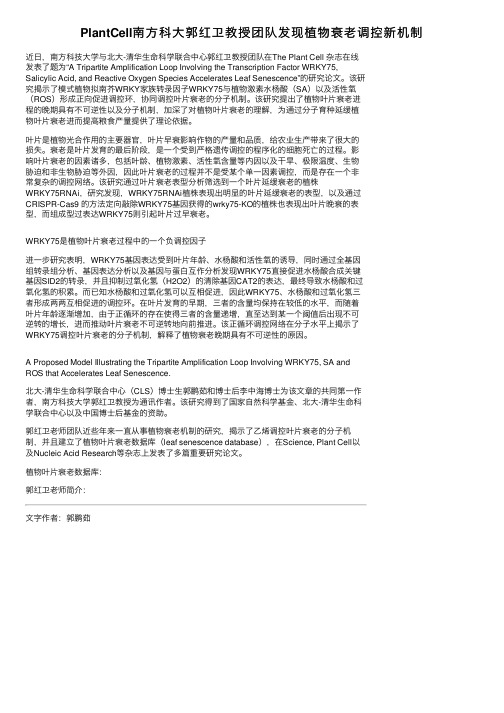
PlantCell南⽅科⼤郭红卫教授团队发现植物衰⽼调控新机制近⽇,南⽅科技⼤学与北⼤-清华⽣命科学联合中⼼郭红卫教授团队在The Plant Cell 杂志在线发表了题为“A Tripartite Amplification Loop Involving the Transcription Factor WRKY75,Salicylic Acid, and Reactive Oxygen Species Accelerates Leaf Senescence”的研究论⽂。
该研究揭⽰了模式植物拟南芥WRKY家族转录因⼦WRKY75与植物激素⽔杨酸(SA)以及活性氧(ROS)形成正向促进调控环,协同调控叶⽚衰⽼的分⼦机制。
该研究提出了植物叶⽚衰⽼进程的晚期具有不可逆性以及分⼦机制,加深了对植物叶⽚衰⽼的理解,为通过分⼦育种延缓植物叶⽚衰⽼进⽽提⾼粮⾷产量提供了理论依据。
叶⽚是植物光合作⽤的主要器官,叶⽚早衰影响作物的产量和品质,给农业⽣产带来了很⼤的损失。
衰⽼是叶⽚发育的最后阶段,是⼀个受到严格遗传调控的程序化的细胞死亡的过程。
影响叶⽚衰⽼的因素诸多,包括叶龄、植物激素、活性氧含量等内因以及⼲旱、极限温度、⽣物胁迫和⾮⽣物胁迫等外因,因此叶⽚衰⽼的过程并不是受某个单⼀因素调控,⽽是存在⼀个⾮常复杂的调控⽹络。
该研究通过叶⽚衰⽼表型分析筛选到⼀个叶⽚延缓衰⽼的植株WRKY75RNAi,研究发现,WRKY75RNAi植株表现出明显的叶⽚延缓衰⽼的表型,以及通过CRISPR-Cas9 的⽅法定向敲除WRKY75基因获得的wrky75-KO的植株也表现出叶⽚晚衰的表型,⽽组成型过表达WRKY75则引起叶⽚过早衰⽼。
WRKY75是植物叶⽚衰⽼过程中的⼀个负调控因⼦进⼀步研究表明,WRKY75基因表达受到叶⽚年龄、⽔杨酸和活性氧的诱导,同时通过全基因组转录组分析、基因表达分析以及基因与蛋⽩互作分析发现WRKY75直接促进⽔杨酸合成关键基因SID2的转录,并且抑制过氧化氢(H2O2)的清除基因CAT2的表达,最终导致⽔杨酸和过氧化氢的积累。
《Plant Cell》杂志:植物科学领域的旗舰
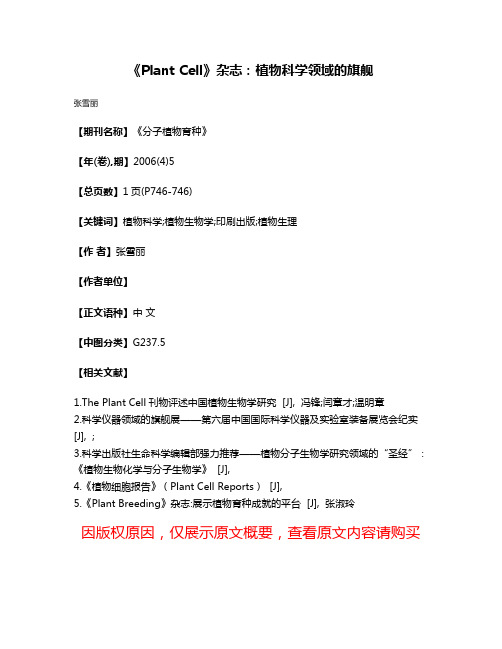
《Plant Cell》杂志:植物科学领域的旗舰
张雪丽
【期刊名称】《分子植物育种》
【年(卷),期】2006(4)5
【总页数】1页(P746-746)
【关键词】植物科学;植物生物学;印刷出版;植物生理
【作者】张雪丽
【作者单位】
【正文语种】中文
【中图分类】G237.5
【相关文献】
1.The Plant Cell刊物评述中国植物生物学研究 [J], 冯锋;闫章才;温明章
2.科学仪器领域的旗舰展——第六届中国国际科学仪器及实验室装备展览会纪实[J], ;
3.科学出版社生命科学编辑部强力推荐——植物分子生物学研究领域的“圣经”:《植物生物化学与分子生物学》 [J],
4.《植物细胞报告》(Plant Cell Reports) [J],
5.《Plant Breeding》杂志:展示植物育种成就的平台 [J], 张淑玲
因版权原因,仅展示原文概要,查看原文内容请购买。
PlantCell背靠背刘宏涛团队、邓兴旺团队揭示植物光形态建成调控新机制
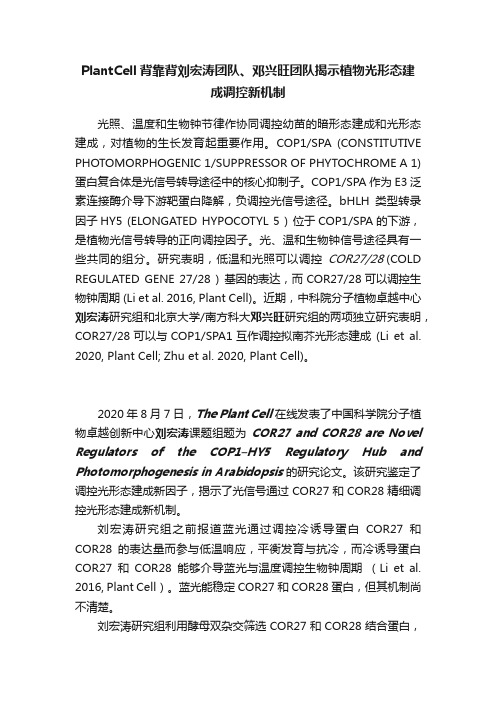
PlantCell背靠背刘宏涛团队、邓兴旺团队揭示植物光形态建成调控新机制光照、温度和生物钟节律作协同调控幼苗的暗形态建成和光形态建成,对植物的生长发育起重要作用。
COP1/SPA (CONSTITUTIVE PHOTOMORPHOGENIC 1/SUPPRESSOR OF PHYTOCHROME A 1) 蛋白复合体是光信号转导途径中的核心抑制子。
COP1/SPA作为E3泛素连接酶介导下游靶蛋白降解,负调控光信号途径。
bHLH类型转录因子HY5 (ELONGATED HYPOCOTYL 5 ) 位于COP1/SPA的下游,是植物光信号转导的正向调控因子。
光、温和生物钟信号途径具有一些共同的组分。
研究表明,低温和光照可以调控COR27/28 (COLD REGULATED GENE 27/28 ) 基因的表达,而COR27/28可以调控生物钟周期 (Li et al. 2016, Plant Cell)。
近期,中科院分子植物卓越中心刘宏涛研究组和北京大学/南方科大邓兴旺研究组的两项独立研究表明,COR27/28可以与COP1/SPA1互作调控拟南芥光形态建成(Li et al. 2020, Plant Cell; Zhu et al. 2020, Plant Cell)。
2020年8月7日,The Plant Cell 在线发表了中国科学院分子植物卓越创新中心刘宏涛课题组题为COR27 and COR28 are Novel Regulators of the COP1–HY5 Regulatory Hub and Photomorphogenesis in Arabidopsis的研究论文。
该研究鉴定了调控光形态建成新因子,揭示了光信号通过COR27和COR28精细调控光形态建成新机制。
刘宏涛研究组之前报道蓝光通过调控冷诱导蛋白COR27和COR28的表达量而参与低温响应,平衡发育与抗冷,而冷诱导蛋白COR27和COR28能够介导蓝光与温度调控生物钟周期(Li et al. 2016, Plant Cell)。
- 1、下载文档前请自行甄别文档内容的完整性,平台不提供额外的编辑、内容补充、找答案等附加服务。
- 2、"仅部分预览"的文档,不可在线预览部分如存在完整性等问题,可反馈申请退款(可完整预览的文档不适用该条件!)。
- 3、如文档侵犯您的权益,请联系客服反馈,我们会尽快为您处理(人工客服工作时间:9:00-18:30)。
Homologous recombination (HR) is essential for maintaining genome integrity and variability. To orchestrate HR in the context of chromatin is a challenge, both in terms of DNA accessibility and restoration of chromatin organization after DNA repair. Histone chaperones function in nucleosome assembly/disassembly and could play a role in HR. Here, we show that the NUCLEOSOME ASSEMBLY PROTEIN1 (NAP1) family histone chaperones are required for somatic HR in Arabidopsis thaliana. Depletion of either the NAP1 group or NAP1-RELATED PROTEIN (NRP) group proteins caused a reduction in HR in plants under normal growth conditions as well as under a wide range of genotoxic or abiotic stresses. This contrasts with the hyperrecombinogenic phenotype caused by the depletion of the CHROMATIN ASSEMBLY FACTOR-1 (CAF-1) histone chaperone. Furthermore, we show that the hyperrecombinogenic phenotype caused by CAF-1 depletion relies on NRP1 and NRP2, but the telomere shortening phenotype does not. Our analysis of DNA lesions, H3K56 acetylation, and expression of DNA repair genes argues for a role of NAP1 family histone chaperones in nucleosome disassembly/reassembly during HR. Our study highlights distinct functions for different families of histone chaperones in the maintenance of genome stability and establishes a crucial function for NAP1 family histone chaperones in somatic HR.
The Plant Cell, Vol. 24: 1437–1447, April 2012, ã 2012 American Society of Plant Biologists. All rights reserved.
Hale Waihona Puke NAP1 Family Histone Chaperones Are Required for Somatic Homologous Recombination in Arabidopsis
INTRODUCTION Homologous recombination (HR) has two key functions in life processes (Schuermann et al., 2005; Heyer et al., 2010). In meiosis, HR is required for accurate chromosome segregation and forms the basis of genetic mixing between maternal and paternal origin chromosomes, thus contributing to novel combinations of genetic traits that enable both natural organism evolution and agricultural breeding programs. In somatic tissues, HR is also important for repairing damaged DNA to maintain genome integrity, hence contributing to the normal growth and development of an organism. The molecular mechanisms that regulate HR at the DNA level for double-strand break (DSB) signaling and repair have been characterized in great detail (Schuermann et al., 2005; Heyer et al., 2010; Knoll and Puchta, 2011), but little is known about HR regulation in the context of chromatin. The basic repeating unit of chromatin is the nucleosome, which consists of 146 bp of DNA wrapped around a histone octamer composed of an (H3-H4)2 tetramer at the center and two H2A-H2B dimers attached symmetrically on either side
1 These
2 Address
authors contributed equally to this work. correspondence to wen-hui.shen@ibmp-cnrs.unistra.fr. The author responsible for distribution of materials integral to the findings presented in this article in accordance with the policy described in the Instructions for Authors () is: Wen-Hui Shen (wen-hui. shen@ibmp-cnrs.unistra.fr). C Some figures in this article are displayed in color online but in black and white in the print edition. W Online version contains Web-only data. /cgi/doi/10.1105/tpc.112.096792
(Luger et al., 1997). Nucleosome compaction limits DNA accessibility to DNA repair proteins, including those involved in HR. Also, proper chromatin structure needs to be restored after DNA repair. Dynamic nucleosome disassembly/reassembly mediated by histone chaperones is thought to play an important role in DNA repair (Ransom et al., 2010; Avvakumov et al., 2011). However, depletion of CHROMATIN ASSEMBLY FACTOR-1 (CAF-1), an evolutionarily conserved histone H3/H4-type chaperone, does not reduce but rather elevates HR in Arabidopsis thaliana plants (Endo et al., 2006; Kirik et al., 2006) as well as in yeast cells (Endo et al., 2010). Although another histone H3/H4type chaperone ANTI-SILENCING FUNCTION1 (ASF1) is required for HR in yeast, this is associated with ASF1 function in histone H3 Lys 56 acetylation (H3K56ac) rather than in physical deposition of histones in nucleosome assembly (Endo et al., 2010; Costelloe and Lowndes, 2010). Therefore, an active role of histone chaperone–mediated nucleosome disassembly/ reassembly in HR remains elusive. NUCLEOSOME ASSEMBLY PROTEIN1 (NAP1) belongs to a family of evolutionarily conserved histone chaperones. NAP1 binds all types of histones in vitro and is found primarily associated with H2A/H2B in vivo and thus is considered as an H2A/ H2B-type histone chaperone (Dong et al., 2003, 2005; Park and Luger, 2006; Zhu et al., 2006). NAP1 proteins are implicated in histone trafficking (Mosammaparast et al., 2002; Miyaji-Yamaguchi et al., 2003; Dong et al., 2005), nucleosome assembly (Ito et al., 1996; Andrews et al., 2010), and disassembly (Lorch et al., 2006; Walfridsson et al., 2007). In Arabidopsis, the NAP1 family comprises four NAP1 genes (NAP1;1, NAP1;2, NAP1;3, and NAP1;4) and two NAP1-RELATED PROTEIN genes (NRP1 and NRP2) (Galichet and Gruissem, 2006; Zhu et al., 2006). Remarkably,