Characterization of a Differential Radio-Frequency Single-Electron Transistor
烟草重要基因篇:3. 烟草烟碱合成代谢相关基因

烟草重要基因篇:3. 烟草烟碱合成代谢相关基因作者:张洪博来源:《中国烟草科学》2014年第03期烟碱(nicotine),即尼古丁,是烟草生物碱(包括烟碱、降烟碱、新烟草碱和假木贼碱等)的一种,约占烟草生物碱总含量的90%~95%[1]。
烟叶烟碱含量占叶片干重的0.6%~3.0%,是烟草和卷烟质量的一项重要指标。
对烟碱代谢的分子遗传学研究可以揭示烟碱代谢累积的分子机制,并为烟碱含量及烟碱成分调节相关的育种工作提供理论基础。
近年来,有关烟碱合成、转运及转化的一些重要基因已被陆续克隆,对烟碱合成代谢机理研究和烟草遗传育种工作产生了重要推动作用。
1 烟碱合成相关基因烟碱分子由一个吡咯烷环和一个吡啶环构成,在烟草根部合成,通过木质部向地上部运输,在烟草植株的叶片中含量最高,茎部含量最低[2-3]。
烟碱吡咯烷环由氮代谢中形成的腐胺合成。
腐胺可通过精氨酸脱羧酶(ADC,arginine decarboxylase)催化精氨酸脱羧形成,或由鸟氨酸脱羧酶(ODC,ornithine decarboxylase)催化鸟氨酸脱羧形成[4-6]。
腐胺在腐胺N-甲基转移酶(PMT,putrescine-N-methyltransferase)作用下获得由S-腺苷蛋氨酸(SAM,S-adenosyl-L-methionine)提供的甲基形成 N-甲基腐胺[7-9],这是一个依赖S-腺苷蛋氨酸合成酶(SAMS,S-adenosylmethionine synthase)活性的反应。
N-甲基腐胺在N-甲基腐胺氧化酶(MPO,N-methylputrescine oxidase)催化下形成4-甲氨基丁醚[10],并通过自身环化形成N-甲基-△1-吡咯啉阳离子,随后与提供吡啶环部分的烟酸衍生物发生缩合反应形成烟碱[11]。
烟碱吡啶环部分由烟酸提供,其前体是由天冬氨酸合成的喹啉酸[12]。
喹啉酸在喹啉酸磷酸核糖转移酶(QPRT,quinolinate phosphoribosyltransferase)催化下形成烟酰胺腺嘌呤二核苷酸(NAD),然后经由吡啶核苷酸循环途径生成烟酸[7,13]。
Materials Characterization
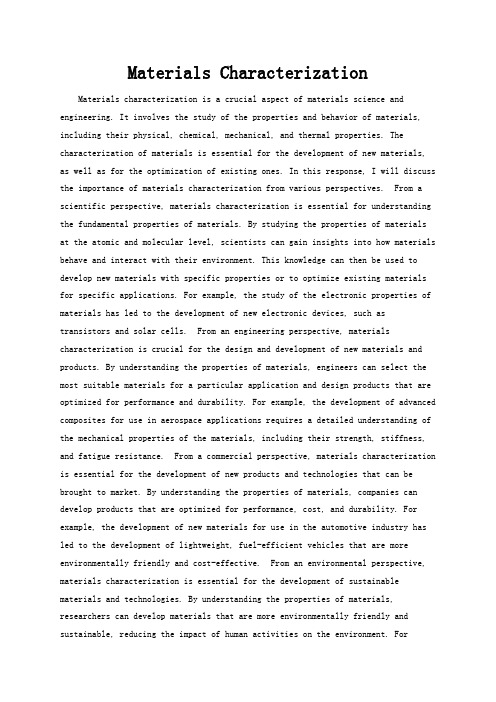
Materials Characterization Materials characterization is a crucial aspect of materials science and engineering. It involves the study of the properties and behavior of materials, including their physical, chemical, mechanical, and thermal properties. The characterization of materials is essential for the development of new materials, as well as for the optimization of existing ones. In this response, I will discuss the importance of materials characterization from various perspectives. From a scientific perspective, materials characterization is essential for understanding the fundamental properties of materials. By studying the properties of materials at the atomic and molecular level, scientists can gain insights into how materials behave and interact with their environment. This knowledge can then be used to develop new materials with specific properties or to optimize existing materials for specific applications. For example, the study of the electronic properties of materials has led to the development of new electronic devices, such astransistors and solar cells. From an engineering perspective, materials characterization is crucial for the design and development of new materials and products. By understanding the properties of materials, engineers can select the most suitable materials for a particular application and design products that are optimized for performance and durability. For example, the development of advanced composites for use in aerospace applications requires a detailed understanding of the mechanical properties of the materials, including their strength, stiffness, and fatigue resistance. From a commercial perspective, materials characterization is essential for the development of new products and technologies that can be brought to market. By understanding the properties of materials, companies can develop products that are optimized for performance, cost, and durability. For example, the development of new materials for use in the automotive industry has led to the development of lightweight, fuel-efficient vehicles that are more environmentally friendly and cost-effective. From an environmental perspective, materials characterization is essential for the development of sustainable materials and technologies. By understanding the properties of materials, researchers can develop materials that are more environmentally friendly and sustainable, reducing the impact of human activities on the environment. Forexample, the development of biodegradable materials for use in packaging and other applications has the potential to reduce the amount of waste generated by human activities. From a societal perspective, materials characterization is essential for the development of new technologies that can improve the quality of life for people around the world. By understanding the properties of materials, researchers can develop new medical devices, renewable energy technologies, and other technologies that can help address some of the world's most pressing problems. For example, the development of new materials for use in medical implants has the potential to improve the quality of life for people with chronic illnesses and injuries. In conclusion, materials characterization is a crucial aspect of materials science and engineering that has implications for science, engineering, commerce, the environment, and society as a whole. By understanding the properties of materials, researchers and engineers can develop new materials and technologies that can improve the quality of life for people around the world. As such, materials characterization will continue to be an essential area of research and development in the years to come.。
单斜相阿利特的晶体结构研究(英文)

Recent work have concerned the identification of stabilized modifications at room temperature due to the importance in the quality control of Portland cement. Differential thermal analysis (DTA) showes the T1–T2 transi-
The objective of this paper was to describe the superstructures of the monoclinic modification of C3S. Some indices for describing the reflections due to the superstructures were proposed to express the orientations and
1 Experimental
The specimens were provided by China Building Materials Academy (CBMA). A selected-area electron diffraction (SAED) technique was applied to record the reflections. A TEM device (Model JEM–2010UHR, JEOL, Tokyo, Japan) equipped with a double tilt goniometry was used to record the HRTEM images at an accelerating voltage of 200 kV. The preparation of specimens for the TEM was performed by a conventional method applied for the observation of ceramic powders except for the dispersion of the specimens in ethanol. Table 1 shows the chemical compositions of the specimens, which was provided by the CBMA. The three specimens are remarked as A, B, and C.
Huang_et_al-2008-Journal_of_Pharmaceutical_Sciences
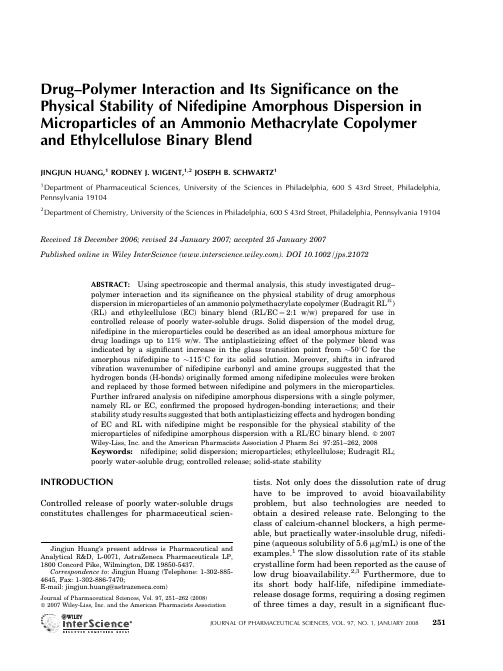
Drug–Polymer Interaction and Its Significance on the Physical Stability of Nifedipine Amorphous Dispersion in Microparticles of an Ammonio Methacrylate Copolymer and Ethylcellulose Binary BlendJINGJUN HUANG,1RODNEY J.WIGENT,1,2JOSEPH B.SCHWARTZ11Department of Pharmaceutical Sciences,University of the Sciences in Philadelphia,600S43rd Street,Philadelphia, Pennsylvania191042Department of Chemistry,University of the Sciences in Philadelphia,600S43rd Street,Philadelphia,Pennsylvania19104Received18December2006;revised24January2007;accepted25January2007Published online in Wiley InterScience().DOI10.1002/jps.21072ABSTRACT:Using spectroscopic and thermal analysis,this study investigated drug–polymer interaction and its significance on the physical stability of drug amorphousdispersion in microparticles of an ammonio polymethacrylate copolymer(Eudragit RL1)(RL)and ethylcellulose(EC)binary blend(RL/EC¼2:1w/w)prepared for use incontrolled release of poorly water-soluble drugs.Solid dispersion of the model drug,nifedipine in the microparticles could be described as an ideal amorphous mixture fordrug loadings up to11%w/w.The antiplasticizing effect of the polymer blend wasindicated by a significant increase in the glass transition point from$508C for theamorphous nifedipine to$1158C for its solid solution.Moreover,shifts in infraredvibration wavenumber of nifedipine carbonyl and amine groups suggested that thehydrogen bonds(H-bonds)originally formed among nifedipine molecules were brokenand replaced by those formed between nifedipine and polymers in the microparticles.Further infrared analysis on nifedipine amorphous dispersions with a single polymer,namely RL or EC,confirmed the proposed hydrogen-bonding interactions;and theirstability study results suggested that both antiplasticizing effects and hydrogen bondingof EC and RL with nifedipine might be responsible for the physical stability of themicroparticles of nifedipine amorphous dispersion with a RL/EC binary blend.ß2007Wiley-Liss,Inc.and the American Pharmacists Association J Pharm Sci97:251–262,2008Keywords:nifedipine;solid dispersion;microparticles;ethylcellulose;Eudragit RL;poorly water-soluble drug;controlled release;solid-state stabilityINTRODUCTIONControlled release of poorly water-soluble drugs constitutes challenges for pharmaceutical scien-tists.Not only does the dissolution rate of drug have to be improved to avoid bioavailability problem,but also technologies are needed to obtain a desired release rate.Belonging to the class of calcium-channel blockers,a high perme-able,but practically water-insoluble drug,nifedi-pine(aqueous solubility of5.6m g/mL)is one of the examples.1The slow dissolution rate of its stable crystalline form had been reported as the cause of low drug bioavailability.2,3Furthermore,due to its short body half-life,nifedipine immediate-release dosage forms,requiring a dosing regimen of three times a day,result in a significantfluc-Jingjun Huang’s present address is Pharmaceutical and Analytical R&D,L-0071,AstraZeneca Pharmaceuticals LP, 1800Concord Pike,Wilmington,DE19850-5437.Correspondence to:Jingjun Huang(Telephone:1-302-885-4645,Fax:1-302-886-7470;E-mail:jingjun.huang@)Journal of Pharmaceutical Sciences,Vol.97,251–262(2008)Pharmacists AssociationJOURNAL OF PHARMACEUTICAL SCIENCES,VOL.97,NO.1,JANUARY2008251tuation of plasma drug concentration that causes toxic side effects.4Consequently,nifedipine con-trolled-release dosage forms have become the practical approach to achieve its therapeutic efficacy through reducing side effects and improv-ing patient compliance.Having potential advantages in achieving a reproducible drug-release rate5and in improving drug bioavailability,6,7microparticle or nanopar-ticle multiparticulate dosage forms may offer a solution to meet the challenges in controlled release of poorly water-soluble drugs.Especially, the matrix-type microparticles containing mole-cularly dispersed drug in water-insoluble poly-meric matrix could potentially improve the dissolution rate of poorly water-soluble drugs and modulate the drug-release rate simulta-neously.Based on this concept,amorphous dis-persion-containing microparticles of hydrophilic and hydrophobic polymer binary blends were pre-viously developed using phase-separation meth-odology for application in controlled delivery of the poorly water-soluble model drug,nifedipine.8,9 Drug-release mechanism and preliminary char-acterization studies on those microparticles indi-cated that due to the formation of an amorphous dispersion,nifedipine release was no longer con-trolled by drug dissolution;but rather it was controlled by the drug diffusion in the matrix.As a result,different combinations of two pharmaceu-tical-grade,insoluble polymers of different hydro-philicity,namely ethylcellulose(EC)and ammonio methacrylate copolymer(Eudragit1RL)(RL), had been demonstrated to be capable to control nifedipine-release rate by changing the drug matrix permeability and microparticle size.More-over,in contrary to the general knowledge that amorphous solid dispersion is inherently ins-table,10it was surprisingly observed that the microparticles,containing$10%of amorphously dispersed nifedipine in a binary blend of RL/ EC¼2:1and1:2w/w,appeared to be stable upon long-term storage.8No significant changes in the drug physical form and drug-release rate were observed on those microparticle samples stored in a closed container after12-month storage at ambient room temperature plus3-month acceler-ated stability at408C/75%relative humidity.In view of those earlier observations,it was of interest to fully characterize the nifedipine solid dispersions formed inside of those microparticles, especially the drug–polymer interactions at the molecular level.An in-depth understanding of the amorphous molecular association between drug and polymers may provide useful informa-tion in understanding how crystallization could be possibly prevented or slowed down by the matrix polymers of this study.Since Fourier-transform infrared spectroscopy(FTIR)has been demonstrated to be capable to detect changes in hydrogen-bonding interactions between func-tional groups as a result of physical form modi-fications,11–13with support of other solid-state characterization techniques including differential scanning calorimeter(DSC)and X-ray powder diffraction spectroscopy(XRPD),infrared spectro-scopy was primarily utilized to investigate the drug–polymer interactions in the microparticles of an RL/EC binary blend.To aid the under-standing of specific drug–polymer interactions, the amorphous dispersions of nifedipine with EC or RL polymer and their physical stability were also evaluated by FTIR.MATERIALSMicronized crystalline nifedipine of stable poly-morph A(NIF)was purchased from Sigma(St. Louis,MO).EC of N7viscosity grade(containing 48.0–49.5w/w of ethoxyl group)was kindly pro-vided by Hercules(Wilmington,DE).Ammonio methacrylate copolymer,Eudragit RL1100(RL) granules(containing6%of molar substitution by the positive-charged quaternary ammonium functional group)were donated by Rohm America (Piscataway,NJ).Acetone and methanol were purchased from Sigma-Aldrich(St.Louis,MO). All other materials were at least of analytical grade.Due to photosensitivity of nifedipine,the storage and handling of drug samples was con-ducted under yellow light.METHODSPreparation of Solid Dispersion-Containing Microparticles by Phase Separation Method Following a phase separation method developed from previous study,8different amounts of nife-dipine(0–3.3g)were dissolved in a50-mL acetone solution containing7.5g of RL and EC polymer blend of a weight ratio of2:1.While the mixture was stirred at600rpm throughout the experi-ment,a volume of100mL purified water(non-solvent)was added dropwise into the drug and polymer solution at a rate1mL/min.In the courseJOURNAL OF PHARMACEUTICAL SCIENCES,VOL.97,NO.1,JANUARY2008DOI10.1002/jps 252HUANG,WIGENT,AND SCHWARTZof the water addition,the drug and polymers were coprecipitated out to form microparticles.At the end of water addition,the solidified micro-particle suspension was then vacuumfiltered with a Whatman#5filter disk,and vacuum dried at room temperature for72h.The dried micropar-ticles were then stored in a desiccator at room temperature and protected from light until use. Preparation of Nifedipine Solid Dispersion With Polymer by Hot-Melt MethodAt a ratio of1:9w/w,nifedipine was mixed with RL or EC polymer to homogeneity in a stainless vessel using a spatula.With nitrogen blanketing over the powder blend inside the vessel,the mixture was heated on a hot plate with tempera-ture controlled at$1768C by a thermostat.After the powder was melted,the vessel was removed from the heat and cooled to room temperature to solidify the comelt;gently ground the comelt with a mortar and pestle for$10–20s;stored the samples in a desiccator at room temperature and protected from light until use.Analysis of Nifedipine ContentFor nifedipine loading determination,micropar-ticles were dissolved in methanol to obtain a theoretical nifedipine concentration of20mg/L. The drug concentration was then analyzed using a UV-VIS spectrophotometer at236nm with a standard curve of bracketed concentrations of nifedipine methanol solution.No interference from the polymers or SDS on the nifedipine assay was found at236nm.Light Microscopic Characterization Microparticles were dispersed in mineral oil on a glass slide and covered with a cover glass.The microparticles were observed under an Olympus polarized light microscope equipped with a digital camera and image analysis software(Image-Pro1 Plus4.5software for Windows TM,San Diego,CA). X-ray Powder Diffraction(XRPD)XRPD2was carried out with a Philips X’Pert powder diffractometer.A CuK a source operation (40kV,50mA)was employed.The diffraction patterns were recorded over a2u angular range of2–408with a step size of0.028in2u and a6s counting per step at room temperature. Differential Scanning Calorimetry(DSC)Thermal analysis was carried out using DSC (Model:DSC2910,TA Instruments Inc.,New Castle,DE).At a heating rate of108C/min,sam-ples of2–5mg were heated from room tempera-ture to2008C in an open aluminum pan under a 10mL/min stream of nitrogen purge.Universal Analysis(Version 2.5)software was used for analysis.Fourier-Transform Infrared(FTIR)The FTIR spectra of samples were obtained,using an FTIR spectrophotometer(Nicolet Magna560, Nicolet Instrument,Madison,WI).About2mg of the samples were mixed with dried potassium bromide of equal weight and compressed to form a KBr disc.The samples were scanned64times from400to4000cmÀ1.RESULTSUsing a phase separation method developed from previous study,8nifedipine microparticles with various drug loading levels,which possessed desired physical properties,such as nearly sphe-rical shape,a narrow size distribution,and a median particle size of$10–20m m,were prepared using the polymer ratio of RL/EC¼2:1w/w.Those microparticles were later used for the character-ization studies.An image of representative micro-particles under a polarized light microscopy is shown in Figure1.Drug and Polymer Crystallinity in Microparticles by XRPDXRPD had been used to identify crystalline phase and to determine drug maximum amorphous drug loading in polymeric matrix at room tempera-ture.14To aid the analysis of drug–polymer interactions of this study,XRPD was used to evaluate the crystallinity of nifedipine,EC,and RL polymers,and to identify possible formation of other nifedipine polymorphs in the microparticles. The X-ray diffractograms of drug-loaded micro-particles together with those of crystalline nife-dipine(stable polymorph A),untreated EC and RL polymers,and plain microparticles without drugDOI10.1002/jps JOURNAL OF PHARMACEUTICAL SCIENCES,VOL.97,NO.1,JANUARY2008DRUG–POLYMER INTERACTION253loading were shown in Figure 2.As indicated by the X-ray diffractograms,RL polymer was an amorphous material in nature,whereas partial crystallinity was observed on untreated EC pow-der.The halo shape of X-ray diffractograms of the plain microparticles indicated that EC lost its partial crystallinity and was completely converted into the amorphous form after microparticle pre-paration.For nifedipine-loaded microparticles with up to21%(w/w)of drug loading,the characteristic peaks of crystalline nifedipine were not detected in the X-ray diffractograms,indicating that nife-dipine was mainly in the amorphous state in those microparticle.When the drug loadings weregreater than or equal to32%w/w,those characteristic peaks of crystalline nifedipine of stable form were observed in the diffractograms. No other nifedipine crystalline polymorphs were observed.Nifedipine–Polymer Interactions in Microparticles by Thermal AnalysisThe melting point of crystalline nifedipine(poly-morph form A)was observed at$1758C(Fig.3). No significant depressing of crystalline nifedipine melting point was observed on the physical mix-ture of crystalline nifedipine and the plain micro-particles of(10%w/w of nifedipine),which showed a melting point of nifedipine at$1748C.The amorphous nifedipine sample prepared using the melting and chilling method described by Forster et al.15was observed to have a glass transition with an enthalpy recovery endotherm at$40–508C,exothermic recrystallization peaks at$88–1308C,and an endothermic melting peak at $1728C.16,17For nifedipine solid dispersion-containing micro-particles,when drug loading was less than or equal to11%(w/w),other than the ECexothermal Figure2.Evaluation of microparticle crystallinity byX-ray powder diffraction.EC,untreated ethylcelluloseN7powder;RL,untreated Eudragit RL powder;%,drugloading(w/w),NIF,crystallinenifedipine.Figure1.Nifedipine microparticles(RL/EC¼2:1w/w)with14%w/w of drug loading by polarized lightmicroscopy(magnification:40Â).Scale bar equals to20mm.Figure3.DSC thermal analysis of nifedipine of dif-ferent physical forms.ANIF,amorphous nifedipine;NIF,crystalline nifedipine;NIFþplain microparticles,the physical mixture of plain microparticles with10%w/w of crystalline nifedipine.JOURNAL OF PHARMACEUTICAL SCIENCES,VOL.97,NO.1,JANUARY2008DOI10.1002/jps 254HUANG,WIGENT,AND SCHWARTZdegradation peak at $160–1808C,18neither amor-phous nifedipine glass transition nor the melting peak of crystalline nifedipine was displayed in the DSC thermograms (Fig.4A).Instead,a weak glass transition attributed to the drug–polymer solid solution was observed,decreasing from $125to $1158C with increasing drug loading from 0%to 11%w/w.The measured T g values of the drug–polymer solid solution were in an agreement with the values estimated by the modified Gordon–Taylor equation developed from an ideal amor-phous solid solution system 19,20(Eq.(1))(Fig.4B).The study result suggested that nifedipine,interacting with polymers as a plasticizer,wasmiscible with polymers in microparticle as an amorphous solid solution.21The significant increase in the glass transition point from $508C for nifedipine amorphous state to $1158C for its solid solution state indicated the antiplas-ticizing effect of the polymer blend on the amorphous dispersed drug:T g12¼w 1T g1þw 2K T g2w 1þK w 2(1)where w 1and w 2are the weight fraction of each component,and T g1and T g2are the corresponding glass transition temperature of each component.For this study,the components 1and 2repre-sented the polymer blend (RL/EC ¼2:1w/w)and amorphous nifedipine,ing Simha–Boyer rule,22the constant K was esti-mated from the glass transition temperature and density of the two components:K ffir 1T g1r 2T g2(2)where T g1and T g2were defined previously,and r 1and r 2are the density of each component.For this study,the T g values of the polymer blend (1258C)and the amorphous nifedipine (508C)were mea-sured by DSC.The density of amorphous nifedi-pine (1.36g/cm 3)was obtained from literature by Forster et al.23;and the density of the polymer blend (0.93g/cm 3)is a weight-averaged value calculated from that of EC (1.13g/cm 3)and RL (0.83g/cm 3).24When the nifedipine loading was increased to 21%(w/w)and above,the glass transition of the drug–polymer solid solution became very weak and no further change in the glass transition of the solid solution was observed.Instead,a ther-mal transition at $508C attributed to the glass transition and enthalpy recovery of the amor-phous nifedipine and a new endothermic peak at $153–1578C were displayed in the DSC thermo-grams (Fig.4A).This deviation of the solid dispersion from ideal amorphous mixture with an appearance of the amorphous nifedipine glass transition may suggest phase separation 20of the amorphous nifedipine from the drug–polymer solid solution at those loading levels (Fig.4B).The endothermic thermal transition at $153–1578C (T m1)was attributed to the melting of solid nifedipine domains that were in close contact with polymers inside of the microparticles;the interactions between drug and polymers may have resulted in this endothermic peak at $153–1578C.16,25,26Figure 4.DSC thermal analysis of nifedipine micro-particles with different drug loadings.(A)DSC thermo-grams of microparticles.(B)Phase diagrams of microparticles estimated from the DSC thermal analy-sis;solid line in the phase diagrams (B)represents the glass transition point (T g )of drug–polymer solid solu-tion predicted by the Gordon–Taylor equation (Eq.(1));ANIF,amorphous nifedipine;NIF,crystalline nifedi-pine;T m1,melting temperature of solid nifedipine domains in microparticles;T m2,melting temperature of crystalline nifedipine outside of microparticles.DOI 10.1002/jpsJOURNAL OF PHARMACEUTICAL SCIENCES,VOL.97,NO.1,JANUARY 2008DRUG–POLYMER INTERACTION 255When nifedipine loading levels were further increased to32%w/w or higher,the amorphous nifedipine glass transition with enthalpy recovery endotherm at$508C,a weak exothermic transi-tion attributed to amorphous nifedipine recrys-tallization at$1008C,16,17and two endothermic peaks at$153–1578C(T m1)and$167–1738C (T m2)were observed in one DSC heating cycle. Since the nifedipine crystals of stable polymorph (form A)outside of microparticles were visually observed and confirmed by X-ray powder diffrac-tograms(Fig.2)for the drug loading levels,the endothermic peak at$167–1738C(T m2)was attributed to the melting of crystalline nifedipine form A outside of the microparticles that had less surface contact with polymers.The depres-sion of drug melting point(T m2)from that of pure crystalline nifedipine(1758C)was attributed to the drug–polymer interactions or the impurity effect by polymers.With increasing drug loading, the melting point(T m2)was elevated to1738C at45%w/w of drug loading(Fig.4)and was similar to that of physical mixture of crystalline nifedipine and plain microparticles(1748C)(Fig.3). Drug–Polymer Interactions in Microparticlesby FTIRBecause the frequency of vibrations within a che-mical structure is very sensitive to how the atomsare arranged and how the atoms interact with neighboring functional groups,not only can FTIR provide a detailedfingerprint of pharmaceutical substances of different physical forms,17but also it can detect changes in hydrogen-bonding pattern of amorphous materials11–13,27that cannot be monitored by DSC and X-ray.The strong infrared absorption of the nifedipine hydrogen-bonding groups,namely the amine and carbonyl groups, makes FTIR especially useful in the detection of the specific drug–polymer hydrogen bond (H-bond)interactions in the microparticles of this study.Illustrated in Figure5are the chemical struc-ture and potential H-bond binding sites of nife-dipine,RL,and EC.The structure of nifedipine illustrates that nifedipine can potentially act as either proton acceptor(through the carbonyl groups,–C–O)or proton donor(through the amine group,–NH).13An X-ray diffraction study conducted on crystalline nifedipine28indicated that the H-bonds are formed via the amine group and one of the carbonyl groups,and the nitro group(–NO2)is not involved in the H-bond interactions.RL polymer monomer has one proton acceptor(carbonyl group,–C–O),whereas EC has hydroxyl groups(–OH)functioning as the proton donor or acceptor.The stretching vibration wavenumbers of the carbonyl group of RL and the hydroxide group of EC were found at$1736 and$3484cmÀ1,respectively(Fig.6B).Both bands located at the same regions were also observed in the spectra of the plain microparticles of RL/EC binary blend(Fig.6A,0%of loading). For nifedipine,shifts in stretching vibration wavenumber of the carbonyl and amine groups as a result of changes in hydrogen-bonding pattern caused by physico-chemical modifications1,13,17,29 are summarized in Table 1.Consistent with literaturefindings,1for both nifedipine crystalline polymorph A and its physical mixture with the plain microparticles8(10%w/w of nifedipine), the hydrogen-bonded nifedipine amine and car-bonyl groups were observed to have strong stretc-hing vibration bands at3332cmÀ1(–NH),and at 1679cmÀ1and1689cmÀ1(–C––O),respectively. Figure5.Chemical structure and proposed hydro-gen-bonding sites between(A)nifedipine and nifedipine;(B)Eudragit RL(R1––CH3,H;R2––CH3,CH2CH3;R3––CH2CH2Nþ(CH3)4ClÀ;R2:R3ffi3:0.2mole/mole)and nifedipine;(C)ethylcellulose and nifedipine;---repre-sents hydrogen bonding between functional groups.JOURNAL OF PHARMACEUTICAL SCIENCES,VOL.97,NO.1,JANUARY2008DOI10.1002/jps 256HUANG,WIGENT,AND SCHWARTZFor amorphous nifedipine prepared using the melting and chilling method described by Forster et al.,15due to irregularity and weakening in hydrogen bonding,13,17,20the stretching vibrationbands of the carbonyl and amine groups became broader and less defined (Fig.6A),reflecting an increase in irregularity of ‘‘long-range order of molecular packing’’of the amorphous substance.30Felodipine,a nifedipine analog belonging to the same dihydropyridine calcium-channel blocker class,was reported to have a nonhydrogen-bonded,‘‘free’’amine vibration band at 3420cm À1.13Nitro-derivative of nifedipine,the dehydrogenated form,was found to have a stretching vibration peak of nonhydrogen-bonded,‘‘free’’carbonyl at 1728cm À1.29Completely replacing the two strong bands caused by the carbonyl groups of crystalline nifedipine at 1679cm À1and 1689cm À1,a new sharp stretching vibration band at 1705cm À1was detected on the nifedipine amorphous dispersion-containing microparticles and its relative inten-sity increased with drug loading for up to 21%w/w (Fig.6A).Since this new stretching vibration band was located between that of the carbonyl group of amorphous nifedipine (1701cm À1)17and that of ‘‘free,’’nonhydrogen-bonded carbonyl group (1728cm À1)29(Tab.1),this peak at 1705cm À1was attributed to the nifedipine carbonyl group that formed H-bonds with polymers.A similar literature value of 1707cm À1had been reported for the stretching vibration of hydrogen-bonded carbonyl group.31As the drug loading level was increased to 21%w/w,the development of a shoulder at the lower wavenumber region of the peak at 1705cm À1suggested the presence of a separate nifedipine phase.Eventually,when drug loading was further increased to 32%w/w,the strong bands characteristic of crystalline nifedi-pine (1679and 1689cm À1)dominated and over-lapped other peaks,indicating the presence of nifedipine crystalline phase.Similarly,as a result of breakage of H-bonds between nifedipine amine and carbonyl groups by molecular dispersion,the changes in nifedi-pine hydrogen-bonding pattern in microparticles were also reflected by concomitant shifts in nife-dipine amine stretching vibration wavenumber (Fig.6A).As the nifedipine loading increased,a new broad band at the higher wavenumber region of $3364cm À1developed in the spectra of nife-dipine amorphous dispersion-containing micro-particles.This peak was most apparent for the microparticles containing 11%w/w of drug load-ing.Since this peak was also located in the region between that of the amine group of amorphous nifedipine (3346cm À1)17and the ‘‘free,’’nonhy-drogen-bonded amine groups (3420cm À1),13thisFigure 6.Investigation of drug–polymer interactions by FTIR.(A)Microparticles of nifedipine solid disper-sion with an EC and RL binary blend (RL/EC ¼2:1)at different drug loadings.(B)Solid dispersion of nifedi-pine with a single polymer RL or EC (10%w/w).NIF,crystalline nifedipine;ANIF,amorphous nifedipine;NIFRL,solid dispersion of nifedipine with RL;NIFEC,solid dispersion of nifedipine with EC;EC,untreated ethylcellulose N7;RL,untreated Eudragit RL.DOI 10.1002/jpsJOURNAL OF PHARMACEUTICAL SCIENCES,VOL.97,NO.1,JANUARY 2008DRUG–POLYMER INTERACTION 257stretching vibration at$3364cmÀ1was postu-lated to be the nifedipine amine group associating with polymers via H-bonds.Illustration of Specific Drug–Polymer Interaction of Nifedipine With EC or RLTo assess the specific interactions between nifedipine and polymers,nifedipine amorphous dispersion with a single polymer,namely EC or RL(10%w/w of nifedipine),were prepared and characterized by FTIR(Fig.6B).To ensure that no solvent or water would interfere the FTIR analysis,these two solid dispersions were pre-pared by hot-melt method.Similar to the nifedipine amorphous dispersion-containing microparticles of RL/EC¼2:1with 11%w/w of drug loading,the stretching vibration bands characteristic of the amine and carbonyl groups of crystalline nifedipine at3332,1689, and1679cmÀ1completely disappeared from the spectra of NIF–RL solid dispersion(Fig.6B), indicating that nifedipine was in an amorphous state in the solid dispersion.The infrared spectra of the NIF–RL solid dispersion also displayed a new broad band at$3374cmÀ1,which,based on the band location(Tab.1),was attributed to the stretching vibration of nifedipine amine groups that formed H-bonds with RL carbonyl groups. However,a difference from that of the nifedipine amorphous dispersion-containing microparticles was observed on the spectra of the NIF–RL solid dispersion.The sharp stretching vibration peak at 1705cmÀ1previously observed on those micro-particles was not found.Instead,only a hump was shown at the lower wavenumber region adjacent to the free RL carbonyl band at$1736cmÀ1.Given the structure of nifedipine and RL polymer (Fig.5B),the abovefindings suggested that the H-bonds formed between the amine and carbonyl groups of crystalline nifedipine may have been disrupted by solid dispersion and been replaced by the H-bonds formed between nifedipine amine and RL carbonyl groups.As a result,the nife-dipine carbonyl groups became‘‘free,’’and its stretching vibration moved a higher wavenumber region($1728cmÀ1),29which,however,was over-lapped by the broad band caused by the free RL carbonyl groups at$1736cmÀ1.On the other hand,since some of the RL carbonyl group formed H-bonds with the nifedipine amine group,the stretching vibration wavenumber of those hydro-gen-bonded RL carbonyl was moved to a lower wavenumber region and was shown as a hump adjacent to the stretching band caused by the ‘‘free’’RL carbonyl group.Similar to the NIF–RL amorphous dispersion and nifedipine amorphous dispersion-containing microparticles,the stretching vibration bands of the amine and carbonyl groups of crystalline nifedipine at3332,1689,and1679cmÀ1were alsoTable1.Shifts of the Stretching Vibration Wavenumber of Carbonyl and Amine Groups at Different FormsFormulations Carbonyl,–C––O(cmÀ1)Amine,–N–H(cmÀ1)Assignment of HydrogenBondingCrystalline nifedipine1679,1679a3331a NIF–C––O---HN–NIF e Physical mixture of microparticles(RL/EC¼2:1)with crystalline NIF(10%w/w of NIF)1679,1689b3332b NIF–C––O---HN–NIF e Amorphous nifedipine1701c3346c NIF–C––O---HN–NIF e Microparticles of RL/EC¼2:1(11%w/w of NIF)1705d3364d NIF–C––O---HO–ECNIF–NH---O––C–RLNIF–NH---O(H)–ECNIF solid dispersion with RL(10%w/w of NIF)—3374NIF–NH---O––C–RLNIF solid dispersion with EC(10%w/w of NIF)17063354NIF–C––O---HO–ECNIF–NH---O(H)–EC‘‘Free’’amine(NIF analog,felodipine e)—3420e Non-H-bonded–NH e‘‘Free’’carbonyl(nifedipine nitro derivative f)1728f—Non-H-bonded–C––ONIF,nifedipine;EC,ethylcellulose;RL,Eudragit1RL;---indicates hydrogen bond interaction.a Ali,1989.1b Huang et al.,2006.8c Burger and Koller,1996.17d Huang et al.,2006.9e Tang et al.,2002.13f Teraoka et al.,1999.29JOURNAL OF PHARMACEUTICAL SCIENCES,VOL.97,NO.1,JANUARY2008DOI10.1002/jps 258HUANG,WIGENT,AND SCHWARTZ。
Characteristics of synchrotron radiation of longitudinally polarized spinning particle in t

·ν
(3)
The second relation in (3) means that the parameter τ becomes a proper time of the particle, while the third relations corresponds to the fact, that the electric dipole moment of the particle qµ is equal to zero, as it should be for a point particle. The first summand in (2) corresponds to the current of the particle without a dipole moment and the second term corresponds to the contribution to the current of the dipole moment of the spinning particle. In the chosen gauge for the theory of the particle without AMM considered here pµν is given by the expression [5]: g · pµν = 2 εµνλσ W λ x σ . (4) m Here W µ denotes the pseudoclassical analog of the Pauli- Lubansky vector, which is connected with the vector of the particle spin in the particle rest frame by the relation Wµ vi (vS ) = γ ( v S ) , Si + γ 2 , m γ+1
1,6-双(N,N′_-二苄基硫代氨基甲酰二硫)己烷的制备及结构表征
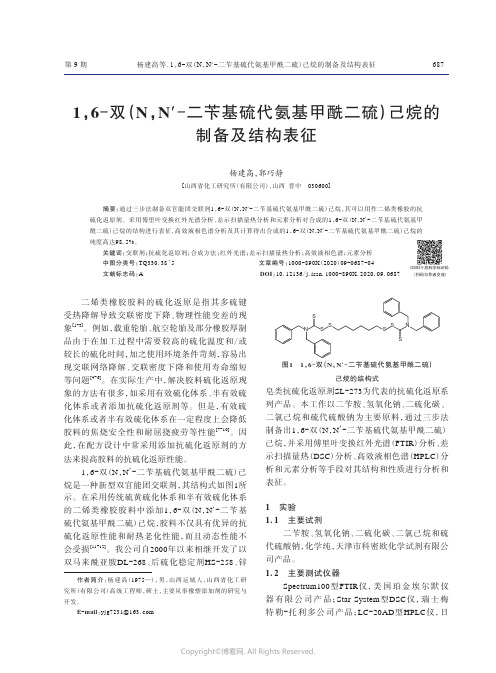
500
400
300
200
100
ֽःϘmV
0
5 10 15 20 25 30 35
ௐᫍmin
1 实验 1. 1 主要试剂
二 苄 胺、氢 氧 化 钠、二 硫 化 碳、二 氯 己 烷 和 硫 代硫酸钠,化学纯,天津市科密欧化学试剂有限公 司产品。 1. 2 主要测试仪器
Spectrum100型FTIR仪,美 国 珀 金 埃 尔 默 仪 器 有 限 公 司 产 品;Star System型DSC仪,瑞 士 梅 特 勒-托 利 多 公 司 产 品;LC-20AD型HPLC仪,日
Copyright©博看网. All Rights Reserved.
688
橡 胶 工 业
2020年第67卷
本岛津公司产品;Vario ELⅢ型元素分析仪,德国 Elementar公司产品。 1. 3 试样制备
将40 g二苄胺、8. 4 g氢氧化钠以及适量的去 离子水加入到四口瓶中,充分搅拌,缓慢滴加15. 2 g二硫化碳,滴加过程中应控制滴加速度并保持反 应温度不超过40 ℃,滴加完毕后反应1 h,得到二 苄 胺 二 硫 代 氨 基 甲 酸 钠 中 间 体 水 溶 液,备 用。 将 15. 5 g二氯己烷和25 g硫代硫酸钠加入到另一个 装 有 去 离 子 水 的 四 口 烧 瓶 中,升 温 至 回 流 状 态 下 反应12 h,得到六亚甲基1,6-二硫代硫酸二钠中 间体水溶液。将第1步制备的二苄胺二硫代氨基 甲酸钠中间体水溶液通过恒压漏斗缓慢滴加入该 反应体系,回流状态下反应1. 5 h,对反应物进行过 滤、水洗、干燥,得到目标产物1,6-双(N,N′ -二苄 基硫代氨基甲酰二硫)己烷。 1. 4 测试表征 1. 4. 1 FTIR分析
常见材料表征技术
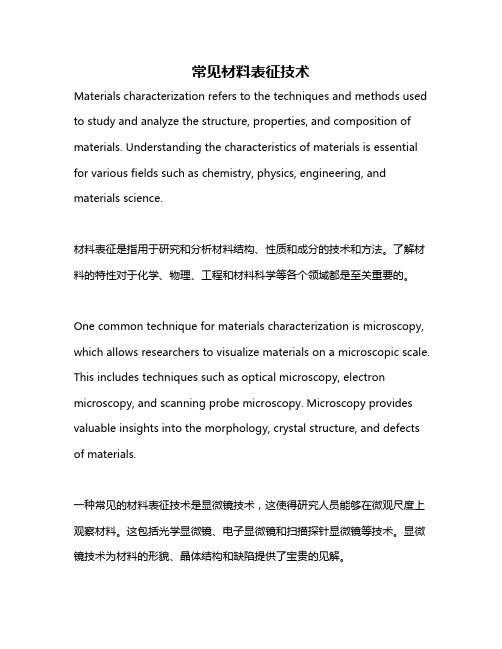
常见材料表征技术Materials characterization refers to the techniques and methods used to study and analyze the structure, properties, and composition of materials. Understanding the characteristics of materials is essential for various fields such as chemistry, physics, engineering, and materials science.材料表征是指用于研究和分析材料结构、性质和成分的技术和方法。
了解材料的特性对于化学、物理、工程和材料科学等各个领域都是至关重要的。
One common technique for materials characterization is microscopy, which allows researchers to visualize materials on a microscopic scale. This includes techniques such as optical microscopy, electron microscopy, and scanning probe microscopy. Microscopy provides valuable insights into the morphology, crystal structure, and defects of materials.一种常见的材料表征技术是显微镜技术,这使得研究人员能够在微观尺度上观察材料。
这包括光学显微镜、电子显微镜和扫描探针显微镜等技术。
显微镜技术为材料的形貌、晶体结构和缺陷提供了宝贵的见解。
Spectroscopy is another important technique for materials characterization. It involves the interaction of materials with different forms of radiation, such as light or X-rays, to study their chemical composition and electronic structure. Spectroscopic techniques, including infrared spectroscopy, Raman spectroscopy, and X-ray photoelectron spectroscopy, provide valuable information about the molecular and electronic properties of materials.光谱学是材料表征的另一个重要技术。
单细胞细胞亚群英文表示
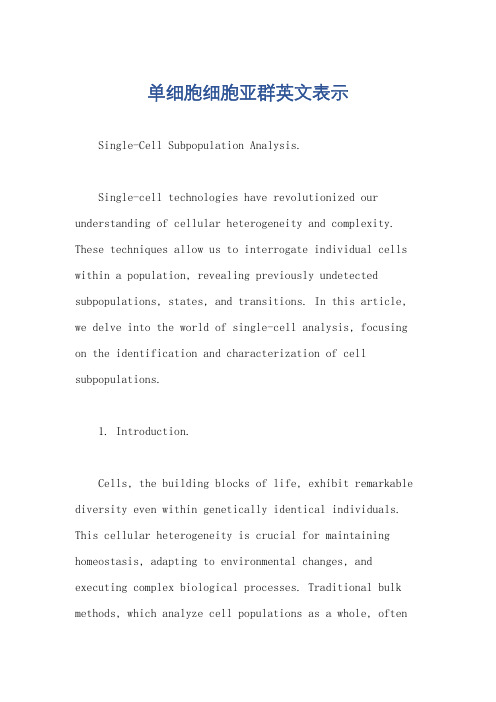
单细胞细胞亚群英文表示Single-Cell Subpopulation Analysis.Single-cell technologies have revolutionized our understanding of cellular heterogeneity and complexity. These techniques allow us to interrogate individual cells within a population, revealing previously undetected subpopulations, states, and transitions. In this article, we delve into the world of single-cell analysis, focusing on the identification and characterization of cell subpopulations.1. Introduction.Cells, the building blocks of life, exhibit remarkable diversity even within genetically identical individuals. This cellular heterogeneity is crucial for maintaining homeostasis, adapting to environmental changes, and executing complex biological processes. Traditional bulk methods, which analyze cell populations as a whole, oftenoverlook this diversity, treating cells as uniform entities. However, with the advent of single-cell technologies, wecan now peer into the intricacies of cellular landscapes, revealing unique subpopulations, rare cell types, and dynamic cellular states.2. Single-Cell Technologies.Single-cell analysis encompasses a wide range of techniques, each tailored to specific research questions. Some of the commonly used single-cell technologies include:Single-Cell RNA Sequencing (scRNA-seq): This technique allows for the measurement of gene expression profiles at the single-cell level. It provides a snapshot of the transcriptome, revealing differential gene expression patterns among cells.Single-Cell ATAC-seq: This method assesses chromatin accessibility at the single-cell level, offering insights into gene regulatory landscapes.Single-Cell Mass Cytometry (CyTOF): This high-throughput technology enables the simultaneous measurement of multiple proteins in single cells, providing a comprehensive proteomic profile.Single-Cell Imaging: Techniques such as confocal microscopy and super-resolution microscopy allow for the visualization of subcellular structures and molecular interactions within single cells.3. Cell Subpopulation Identification.Single-cell data analysis often involves the identification and characterization of distinct subpopulations within a heterogeneous cell population. This can be achieved through various computational methods, including:Clustering Algorithms: Unsupervised learning algorithms such as k-means, hierarchical clustering, and density-based spatial clustering of applications with noise (DBSCAN) are commonly used to group cells based on theirsimilarity in gene expression, chromatin accessibility, or proteomic profiles. These clusters often correspond to distinct cell types or states.Trajectory Analysis: Methods like Monocle and Scanpy's pseudotime analysis allow for the reconstruction ofcellular trajectories, revealing the sequential order of cellular states during development or differentiation.Differential Expression Analysis: By comparing gene expression profiles between clusters or along pseudotime trajectories, researchers can identify genes that are differentially expressed, thus characterizing the unique features of each subpopulation.4. Applications of Single-Cell Subpopulation Analysis.Single-cell subpopulation analysis has found applications in various fields of biology and medicine, including:Developmental Biology: Studying the dynamics of cellsubpopulations during embryogenesis and organogenesisoffers insights into the mechanisms of cellular specialization and tissue formation.Immunology: Analyzing immune cell subpopulations can reveal the complexity of immune responses and identify novel targets for immunotherapy.Cancer Research: Single-cell analysis of tumor cells canuncover intratumoral heterogeneity, identify cancer stem cells, and elucidate drug resistance mechanisms.Neuroscience: Studying neuronal subpopulations can provide insights into the organization and function of the brain, as well as the pathophysiology of neurological diseases.5. Challenges and Future Directions.While single-cell technologies have revolutionized our understanding of cellular heterogeneity, they also pose several challenges. One of the main limitations is thenoise and technical variation introduced during the experimental and analytical processes. Advanced statistical methods and computational tools are needed to account for these variations and improve the accuracy of subpopulation identification.Moreover, single-cell data analysis often requires extensive computational resources and expertise, limiting its accessibility to a broader research community. Future efforts should focus on developing user-friendly tools and platforms that enable even non-experts to perform single-cell analysis.Despite these challenges, the future of single-cell subpopulation analysis looks bright. With the continuous improvement of experimental techniques and computational methods, we can expect more precise and comprehensive characterizations of cell subpopulations, leading to deeper insights into the complexity of biological systems.In conclusion, single-cell subpopulation analysis has emerged as a powerful tool for studying cellularheterogeneity. By combining experimental techniques with computational methods, we can identify and characterize unique cell subpopulations, revealing the rich diversity and dynamics of cellular landscapes. As the field continues to evolve, we look forward to even more insights into the wonders of cellular biology.。
(完整版)电子信息与通信工程专业英语期末必考翻译
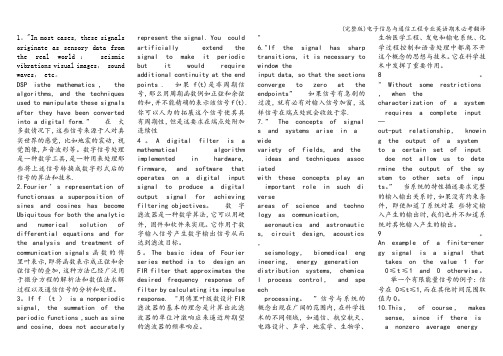
1。
"In most cases, these signals originate as sensory data from the real world:seismic vibrations visual images, sound waves, etc。
DSP isthe mathematics,the algorithms, and the techniques used to manipulate these signals after they have been converted into a digital form.”在大多数情况下,这些信号来源于人对真实世界的感觉,比如地震的震动,视觉图像,声音波形等。
数字信号处理是一种数学工具,是一种用来处理那些将上述信号转换成数字形式后的信号的算法和技术.2.Fourier’s representation of functionsas a superposition of sines and cosines has become Ubiquitous for both the analytic and numerical solution of differential equations and for the analysis and treatment of communication signals 函数的傅里叶表示,即将函数表示成正弦和余弦信号的叠加,这种方法已经广泛用于微分方程的解析法和数值法求解过程以及通信信号的分析和处理。
3。
If f (t ) is a nonperiodic signal, the summation of the periodic functions ,such as sine and cosine, does not accurately represent the signal. You couldartificially extend thesignal to make it periodicbut it would requireadditional continuity at the endpoints . 如果f(t)是非周期信号,那么用周期函数例如正弦和余弦的和,并不能精确的表示该信号f(t).你可以人为的拓展这个信号使其具有周期性,但是这要求在端点处附加连续性4。
一种用于差、共模噪声抑制的平面EMI扼流圈
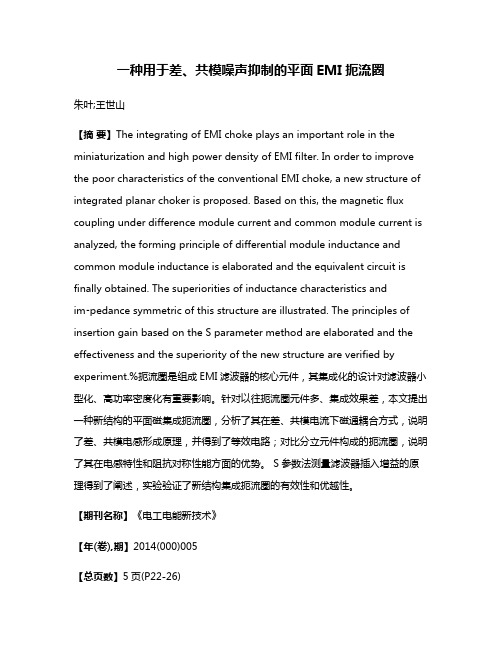
一种用于差、共模噪声抑制的平面EMI扼流圈朱叶;王世山【摘要】The integrating of EMI choke plays an important role in the miniaturization and high power density of EMI filter. In order to improve the poor characteristics of the conventional EMI choke, a new structure of integrated planar choker is proposed. Based on this, the magnetic flux coupling under difference module current and common module current is analyzed, the forming principle of differential module inductance and common module inductance is elaborated and the equivalent circuit is finally obtained. The superiorities of inductance characteristics andim⁃pedance symmetric of this structure are illustrated. The principles of insertion gain based on the S parameter method are elaborated and the effectiveness and the superiority of the new structure are verified by experiment.%扼流圈是组成EMI滤波器的核心元件,其集成化的设计对滤波器小型化、高功率密度化有重要影响。
CrystGrowthDes晶体生长英文文献

An Infinite Two-Dimensional Hybrid Water-Chloride Network,Self-Assembled in a Hydrophobic Terpyridine Iron(II)MatrixRicardo R.Fernandes,†Alexander M.Kirillov,†M.Fátima C.Guedes da Silva,†,‡Zhen Ma,†JoséA.L.da Silva,†João J.R.Fraústo da Silva,†andArmando J.L.Pombeiro*,†Centro de Química Estrutural,Complexo I,Instituto Superior Técnico,TU-Lisbon,A V.Ro V isco Pais,1049-001Lisbon,Portugal,and Uni V ersidade Luso´fona de Humanidades e Tecnologias,A V.doCampo Grande,376,1749-024,Lisbon,PortugalRecei V ed October18,2007;Re V ised Manuscript Recei V ed January7,2008ABSTRACT:An unprecedented two-dimensional water-chloride anionic{[(H2O)20(Cl)4]4–}n network has been structurally identified in a hydrophobic matrix of the iron(II)compound[FeL2]Cl2·10H2O(L)4′-phenyl-2,2′:6′,2″-terpyridine).Its intricate relief geometry has been described as a set of10nonequivalent alternating cycles of different sizes ranging from tetra-to octanuclear{[(H2O)x(Cl)y]y–}z(x) 2–6,y)0–2,z)4–6,8)fragments.In contrast to the blooming research on structural characterizationof a wide variety of water clusters in different crystalline materials,1much less attention has been focused on the identification anddescription of hybrid hydrogen-bonded water assemblies with othersolvents,small molecules,or counterions.1c,2In particular,thecombination of chloride ions and water is one of the most commonlyfound in natural environments(e.g.,seawater or sea-salt aerosols),and thus the investigation of water-chloride interactions has beenthe object of numerous theoretical studies.3However,only recentlya few water-chloride associates incorporated in various crystalmatrixes have been identified and structurally characterized,4,5including examples of(i)discrete cyclic[(H2O)4(Cl)]–,4a[(H2O)4(Cl)2]2–,4b and[(H2O)6(Cl)2]2–4c clusters,and(ii)variousone-or two-dimensional(1D or2D)hydrogen-bonded networksgenerated from crystallization water and chloride counterionswith{[(H2O)4(Cl2)]2–}n,5b{[(H2O)6(Cl)2]2–}n,5b[(H2O)7(HCl)2]n,5c{[(H2O)11(Cl)7]7–}n,5d{[(H2O)14(Cl)2]2–}n,5e{[(H2O)14(Cl)4]4–}n,5aand{[(H2O)14(Cl)5]5–}n5f compositions.These studies are alsobelieved to provide a contribution toward the understanding of thehydration phenomena of chloride ions in nature and have importancein biochemistry,catalysis,supramolecular chemistry,and designof crystalline materials.5In pursuit of our interest in the self-assembly synthesis andcrystallization of various transition metal compounds in aqueousmedia,we have recently described the[(H2O)10]n,6a(H2O)6,6b and[(H2O)4(Cl)2]2–4b clusters hosted by Cu/Na or Ni metal-organicmatrixes.Continuing this research,we report herein the isolationand structural characterization of a unique2D water-chlorideanionic layer{[(H2O)20(Cl)4]4–}n within the crystal structure of thebis-terpyridine iron(II)compound[FeL2]Cl2·10H2O(1′)(L)4′-phenyl-2,2′:6′,2″-terpyridine).Although this compound has beenobtained unexpectedly,a search in the Cambridge StructuralDatabase(CSD)7,8points out that various terpyridine containinghosts tend to stabilize water-chloride associates,thus also sup-porting the recognized ability of terpyridine ligands in supra-molecular chemistry and crystal engineering.9,10Hence,the simple combination of FeCl2·2H2O and L in tetrahydrofuran(THF)solution at room temperature provides the formation of a deep purple solid formulated as[FeL2]Cl2·FeCl2·5H2O(1)on the basis of elemental analysis,FAB+-MS and IR spectroscopy.11This compound reveals a high affinity for water and,upon recrystallization from a MeOH/H2O(v/v)9/1)mixture,leads to single crystals of1′with a higher water content,which have been characterized by single-crystal X-ray analysis.12The asymmetric unit of1′is composed of a cationic[FeL2]2+ part,two chloride anions,and10independent crystallization water molecules(with all their H atoms located in the difference Fourier map),the latter occupying a considerable portion of the crystal cell. The iron atom possesses a significantly distorted octahedral coordination environmentfilled by two tridentate terpyridine moieties arranged in a nearly perpendicular fashion(Figure S1, Supporting Information).Most of the bonding parameters within [FeL2]2+are comparable to those reported for other iron compounds*To whom correspondence should be sent.Fax:+351-21-8464455.E-mail: pombeiro@ist.utl.pt.†Instituto Superior Técnico.‡Universidade Luso´fona de Humanidades eTecnologias.Figure 1.Perspective representations(arbitrary views)of hybrid water-chloride hydrogen-bonded assemblies in the crystal cell of1′; H2O molecules and chloride ions are shown as colored sticks and balls, respectively.(a)Minimal repeating{[(H2O)20(Cl)4]4–}n fragment with atom numbering scheme.(b)Nonplanar infinite polycyclic2D anionic layer generated by linkage of four{[(H2O)20(Cl)4]4–}n fragments(a) represented by different colors;the numbers are those of Table1and define the10nonequivalent alternating cycles of different size.2008310.1021/cg7010315CCC:$40.75 2008American Chemical SocietyPublished on Web02/08/2008bearing two terpyridine ligands.13The most interesting feature of the crystal structure of 1′consists in the extensive hydrogen bonding interactions of all the lattice–water molecules and chloride coun-terions (Table S1,Supporting Information),leading to the formation of a hybrid water -chloride polymeric assembly possessing minimal repeating {[(H 2O)20(Cl)4]4–}n fragments (Figure 1a).These are further interlinked by hydrogen bonds generating a nonplanar 2D water -chloride anionic layer (Figure 1b).Hence,the multicyclic {[(H 2O)20(Cl)4]4–}n fragment is con-structed by means of 12nonequivalent O–H ···O interactions with O ···O distances ranging from 2.727to 2.914Åand eight O–H ···Cl hydrogen bonds with O ···Cl separations varying in the 3.178–3.234Årange (Table S1,Supporting Information).Both average O ···O [∼2.82Å]and O ···Cl [∼3.20Å]separations are comparable to those found in liquid water (i.e.,2.85Å)14and various types of H 2O clusters 1,6or hybrid H 2O -Cl associates.4,5Eight of ten water molecules participate in the formation of three hydrogen bonds each (donating two and accepting one hydrogen),while the O3and O7H 2O molecules along with both Cl1and Cl2ions are involved in four hydrogen-bonding contacts.The resulting 2D network can be considered as a set of alternating cyclic fragments (Figure 1b)which are classified in Table 1and additionally shown by different colors in Figure 2.Altogether there are 10different cycles,that is,five tetranuclear,three pentanuclear,one hexanuclear,and one octa-nuclear fragment (Figures 1b and 2,Table 1).Three of them (cycles 1,2,and 6)are composed of only water molecules,whereas the other seven rings are water -chloride hybrids with one or two Cl atoms.The most lengthy O ···O,O ···Cl,or Cl ···Cl nonbonding separations within rings vary from 4.28to 7.91Å(Table 1,cycles 1and 10,respectively).Most of the cycles are nonplanar (except those derived from the three symmetry generated tetrameric fragments,cycles 1,2,and 4),thus contributing to the formation of an intricate relief geometry of the water -chloride layer,possessing average O ···O ···O,O ···Cl ···O,and O ···O ···Cl angles of ca.104.9,105.9,and 114.6°,respectively (Table S2,Supporting Information).The unprecedented character of thewater -chloride assembly in 1′has been confirmed by a thorough search in the CSD,7,15since the manual analysis of 156potentially significant entries with the minimal [(H 2O)3(Cl)]–core obtained within the searching algorithm 15did not match a similar topology.Nevertheless,we were able to find several other interesting examples 16of infinite 2D and three-dimensional (3D)water -chloride networks,most of them exhibiting strong interactions with metal -organic matrixes.The crystal packing diagram of 1′along the a axis (Figure 3)shows that 2D water -chloride anionic layers occupy the free space between hydrophobic arrays of metal -organic units,with an interlayer separation of 12.2125(13)Åthat is equivalent to the b unit cell dimension.12In contrast to most of the previously identified water clusters,1,6water -chloride networks,5,16and extended assemblies,1c the incorporation of {[(H 2O)20(Cl)4]4–}n sheets in 1′is not supported by strong intermolecular interactions with the terpyridine iron matrix.Nevertheless,four weak C–H ···O hydrogen bonds [avg d (D ···A))3.39Å]between some terpyridine CH atoms and lattice–water molecules (Table S1,Figure S2,Supporting Information)lead to the formation of a 3D supramolecular framework.The thermal gravimetric analysis (combined TG-DSC)of 117(Figure S3,Supporting Information)shows the stepwise elimination of lattice–water in the broad 50–305°C temperature interval,in accord with the detection on the differential scanning calorimetryTable 1.Description of Cyclic Fragments within the {[(H 2O)20(Cl)4]4–}n Network in 1′entry/cycle numbernumber of O/Cl atomsformula atom numberingschemegeometry most lengthy separation,Åcolor code a 14(H 2O)4O3–O4–O3–O4planar O3···O3,4.28light brown 24(H 2O)4O6–O7–O6–O7planar O7···O7,4.42light gray 34[(H 2O)3(Cl)]-O2–O4–O3–Cl2nonplanar O4···Cl2,4.66blue 44[(H 2O)3(Cl)]-O6–O7–O9–Cl1nonplanar O7···Cl1,4.61green 54[(H 2O)2(Cl)2]2-O9–Cl1–O9–Cl1planar Cl ···Cl1,4.76pink 65(H 2O)5O2–O4–O3–O10–O8nonplanar O2···O10,4.55red75[(H 2O)4(Cl)]-O1–O5–O7–O9–Cl1nonplanar O7···Cl1,5.25pale yellow 85[(H 2O)4(Cl)]-O1–O5–Cl2–O8–O10nonplanar O10···Cl2,5.29orange 96[(H 2O)4(Cl)2]2-O2–O8–Cl2–O2–O8–Cl2nonplanar Cl2···Cl2,7.12yellow 108[(H 2O)6(Cl)2]2-O1–O10–O3–Cl2–O5–O7–O6–Cl1nonplanarCl1···Cl2,7.91pale blueaColor codes are those of Figure 2.Figure 2.Fragment of nonplanar infinite polycyclic 2D anionic layer in the crystal cell of 1′.The 10nonequivalent alternating water or water -chloride cycles are shown by different colors (see Table 1for color codes).Figure 3.Fragment of the crystal packing diagram of 1′along the a axis showing the intercalation of two water -chloride layers (represented by space filling model)into the metal -organic matrix (depicted as sticks);color codes within H 2O -Cl layers:O red,Cl green,H grey.Communications Crystal Growth &Design,Vol.8,No.3,2008783curve(DSC)of three major endothermic processes in ca.50–170, 170–200,and200–305°C ranges with maxima at ca.165,190, and280°C,corresponding to the stepwise loss of ca.two,one, and two H2O molecules,respectively(the overall mass loss of9.1% is in accord with the calculated value of9.4%for the elimination of allfive water molecules).In accord,the initial broad and intense IRν(H2O)andδ(H2O)bands of1(maxima at3462and1656cm–1, respectively)gradually decrease in intensity on heating the sample up to ca.305°C,while the other bands remain almost unchangeable. Further heating above305°C leads to the sequential decomposition of the bis-terpyridine iron unit.These observations have also been supported by the IR spectra of the products remaining after heating the sample at different temperatures.The elimination of the last portions of water in1at temperatures as high as250–305°C is not commonly observed(although it is not unprecedented18)for crystalline materials with hosted water clusters,and can be related to the presence and extensive hydrogen-bonding of chloride ions in the crystal cell,tending to form the O–H(water)···Cl hydrogen bonds ca.2.5times stronger in energy than the corresponding O–H(water)···O(water)ones.5a The strong binding of crystallization water in1is also confirmed by its FAB+-MS analysis that reveals the rather uncommon formation of the fragments bearing from one tofive H2O molecules.11The exposure to water vapors for ca.8h of an almost completely dehydrated(as confirmed by weighing and IR spectroscopy)product after thermolysis of1(at250°C19for 30min)results in the reabsorption of water molecules giving a material with weight and IR spectrum identical to those of the initial sample1,thus corroborating the reversibility of the water escape and binding process.In conclusion,we have synthesized and structurally characterized a new type of2D hybrid water-chloride anionic multicyclic {[(H2O)20(Cl)4]4–}n network self-assembled in a hydrophobic matrix of the bis-terpyridine iron(II)complex,that is,[FeL2]Cl2·10H2O 1′.On the basis of the recent description and detailed analysis of the related{[(H2O)14(Cl)4]4–}n layers5a and taking into consideration that the water-chloride assembly in1′does not possess strong interactions with the metal-organic units,the crystal structure of 1′can alternatively be defined as an unusual set of water-chloride “hosts”with bis-terpyridine iron“guests”.Moreover,the present study extends the still limited number5of well-identified examples of large polymeric2D water-chloride assemblies intercalated in crystalline materials and shows that terpyridine compounds can provide rather suitable matrixes to stabilize and store water-chloride aggregates.Further work is currently in progress aiming at searching for possible applications in nanoelectrical devices,as well as understanding how the modification of the terpyridine ligand or the replacement of chlorides by other counterions with a high accepting ability toward hydrogen-bonds can affect the type and topology of the hybrid water containing associates within various terpyridine transition metal complexes.Acknowledgment.This work has been partially supported by the Foundation for Science and Technology(FCT)and its POCI 2010programme(FEDER funded),and by a HRTM Marie Curie Research Training Network(AQUACHEM project,CMTN-CT-2003-503864).The authors gratefully acknowledge Prof.Maria Filipa Ribeiro for kindly running the TG-DSC analysis,urent Benisvy,Dr.Maximilian N.Kopylovich,and Mr.Yauhen Y. Karabach for helpful discussions.Supporting Information Available:Additionalfigures(Figures S1–S3)with structural fragments of1′and TG-DSC analysis of1, Tables S1and S2with hydrogen-bond geometry in1′and bond angles within the H2O-Cl network,details for the general experimental procedures and X-ray crystal structure analysis and refinement,crystal-lographic informationfile(CIF),and the CSD refcodes for terpyridine compounds with water-chloride aggregates.This information is available free of charge via the Internet at .References(1)(a)Mascal,M.;Infantes,L.;Chisholm,J.Angew.Chem.,Int.Ed.2006,45,32and references therein.(b)Infantes,L.;Motherwell,S.CrystEngComm2002,4,454.(c)Infantes,L.;Chisholm,J.;Mother-well,S.CrystEngComm2003,5,480.(d)Supriya,S.;Das,S.K.J.Cluster Sci.2003,14,337.(2)(a)Das,M.C.;Bharadwaj,P.K.Eur.J.Inorg.Chem.2007,1229.(b)Ravikumar,I.;Lakshminarayanan,P.S.;Suresh,E.;Ghosh,P.Cryst.Growth Des.2006,6,2630.(c)Ren,P.;Ding,B.;Shi,W.;Wang,Y.;Lu,T.B.;Cheng,P.Inorg.Chim.Acta2006,359,3824.(d)Li,Z.G.;Xu,J.W.;Via,H.Q.;Hu,mun.2006,9,969.(e)Lakshminarayanan,P.S.;Kumar,D.K.;Ghosh,P.Inorg.Chem.2005,44,7540.(f)Raghuraman,K.;Katti,K.K.;Barbour,L.J.;Pillarsetty,N.;Barnes,C.L.;Katti,K.V.J.Am.Chem.Soc.2003,125,6955.(3)(a)Jungwirth,P.;Tobias,D.J.J.Phys.Chem.B.2002,106,6361.(b)Tobias,D.J.;Jungwirth,P.;Parrinello,M.J.Chem.Phys.2001,114,7036.(c)Choi,J.H.;Kuwata,K.T.;Cao,Y.B.;Okumura,M.J.Phys.Chem.A.1998,102,503.(d)Xantheas,S.S.J.Phys.Chem.1996,100,9703.(e)Markovich,G.;Pollack,S.;Giniger,R.;Cheshnovsky,O.J.Chem.Phys.1994,101,9344.(f)Combariza,J.E.;Kestner,N.R.;Jortner,J.J.Chem.Phys.1994,100,2851.(g)Perera, L.;Berkowitz,M.L.J.Chem.Phys.1991,95,1954.(h)Dang,L.X.;Rice,J.E.;Caldwell,J.;Kollman,P.A.J.Am.Chem.Soc.1991, 113,2481.(4)(a)Custelcean,R.;Gorbunova,M.G.J.Am.Chem.Soc.2005,127,16362.(b)Kopylovich,M.N.;Tronova,E.A.;Haukka,M.;Kirillov,A.M.;Kukushkin,V.Yu.;Fraústo da Silva,J.J.R.;Pombeiro,A.J.L.Eur.J.Inorg.Chem.2007,4621.(c)Butchard,J.R.;Curnow,O.J.;Garrett,D.J.;Maclagan,R.G.A.R.Angew.Chem.,Int.Ed.2006, 45,7550.(5)(a)Reger,D.L.;Semeniuc,R.F.;Pettinari,C.;Luna-Giles,F.;Smith,M.D.Cryst.Growth.Des.2006,6,1068and references therein.(b) Saha,M.K.;Bernal,mun.2005,8,871.(c) Prabhakar,M.;Zacharias,P.S.;Das,mun.2006,9,899.(d)Lakshminarayanan,P.S.;Suresh,E.;Ghosh,P.Angew.Chem.,Int.Ed.2006,45,3807.(e)Ghosh,A.K.;Ghoshal,D.;Ribas,J.;Mostafa,G.;Chaudhuri,N.R.Cryst.Growth.Des.2006,6,36.(f)Deshpande,M.S.;Kumbhar,A.S.;Puranik,V.G.;Selvaraj, K.Cryst.Growth Des.2006,6,743.(6)(a)Karabach,Y.Y.;Kirillov,A.M.;da Silva,M.F.C.G.;Kopylovich,M.N.;Pombeiro,A.J.L.Cryst.Growth Des.2006,6,2200.(b) Kirillova,M.V.;Kirillov,A.M.;da Silva,M.F.C.G.;Kopylovich, M.N.;Fraústo da Silva,J.J.R.;Pombeiro,A.J.L.Inorg.Chim.Acta2008,doi:10.1016/j.ica.2006.12.016.(7)The Cambridge Structural Database(CSD).Allen, F.H.ActaCrystallogr.2002,B58,380.(8)The searching algorithm in the ConQuest Version1.9(CSD version5.28,August2007)constrained to the presence of any terpyridinemoiety and at least one crystallization water molecule and one chloride counter ion resulted in43analyzable hits from which40compounds contain diverse water-chloride aggregates(there are29and11 examples of infinite(mostly1D)networks and discrete clusters, respectively).See the Supporting Information for the CSD refcodes.(9)For a recent review,see Constable,E.C.Chem.Soc.Re V.2007,36,246.(10)For recent examples of supramolecular terpyridine compounds,see(a)Beves,J.E.;Constable,E.C.;Housecroft,C.E.;Kepert,C.J.;Price,D.J.CrystEngComm2007,9,456.(b)Zhou,X.-P.;Ni,W.-X.;Zhan,S.-Z.;Ni,J.;Li,D.;Yin,Y.-G.Inorg.Chem.2007,46,2345.(c)Shi,W.-J.;Hou,L.;Li,D.;Yin,Y.-G.Inorg.Chim.Acta2007,360,588.(d)Beves,J.E.;Constable,E.C.;Housecroft,C.E.;Kepert,C.J.;Neuburger,M.;Price,D.J.;Schaffner,S.CrystEngComm2007,9,1073.(e)Beves,J. E.;Constable, E. C.;Housecroft, C. E.;Neuburger,M.;Schaffner,mun.2007,10,1185.(f)Beves,J.E.;Constable,E.C.;Housecroft,C.E.;Kepert,C.J.;Price,D.J.CrystEngComm2007,9,353.(11)Synthesis of1:FeCl2·2H2O(82mg,0.50mmol)and4′-phenyl-2,2′:6′,2″-terpyridine(L)(154mg,0.50mmol)were combined in a THF (20mL)solution with continuous stirring at room temperature.The resulting deep purple suspension was stirred for1h,filtered off,washed with THF(3×15mL),and dried in vacuo to afford a deep purple solid1(196mg,41%).1exhibits a high affinity for water and upon recrystallization gives derivatives with a higher varying content of crystallization water.1is soluble in H2O,MeOH,EtOH,MeCN, CH2Cl2,and CHCl3.mp>305°C(dec.).Elemental analysis.Found: C52.96,H3.76,N8.36.Calcld.for C42H40Cl4Fe2N6O5:C52.42,H4.19,N8.73.FAB+-MS:m/z:835{[FeL2]Cl2·5H2O+H}+,816784Crystal Growth&Design,Vol.8,No.3,2008Communications{[FeL2]Cl2·4H2O}+,796{[FeL2]Cl2·3H2O–2H}+,781{[FeL2]Cl2·2H2O+H}+,763{[FeL2]Cl2·H2O+H}+,709{[FeL2]Cl}+,674 {[FeL2]}+,435{[FeL]Cl2}+,400{[FeL]Cl}+,364{[FeL]–H}+,311 {L–2H}+.IR(KBr):νmax/cm–1:3462(m br)ν(H2O),3060(w),2968 (w)and2859(w)ν(CH),1656(m br)δ(H2O),1611(s),1538(w), 1466(m),1416(s),1243(m),1159(w),1058(m),877(s),792(s), 766(vs),896(m),655(w),506(m)and461(m)(other bands).The X-ray quality crystals of[FeL2]Cl2·10H2O(1′)were grown by slow evaporation,in air at ca.20°C,of a MeOH/H2O(v/v)9/1)solution of1.(12)Crystal data:1′:C42H50Cl2FeN6O10,M)925.63,triclinic,a)10.1851(10),b)12.2125(13),c)19.5622(19)Å,R)76.602(6),)87.890(7),γ)67.321(6)°,U)2180.3(4)Å3,T)150(2)K,space group P1j,Z)2,µ(Mo-K R))0.532mm-1,32310reflections measured,8363unique(R int)0.0719)which were used in all calculations,R1)0.0469,wR2)0.0952,R1)0.0943,wR2)0.1121 (all data).(13)(a)McMurtrie,J.;Dance,I.CrystEngComm2005,7,230.(b)Nakayama,Y.;Baba,Y.;Yasuda,H.;Kawakita,K.;Ueyama,N.Macromolecules2003,36,7953.(c)Kabir,M.K.;Tobita,H.;Matsuo,H.;Nagayoshi,K.;Yamada,K.;Adachi,K.;Sugiyama,Y.;Kitagawa,S.;Kawata,S.Cryst.Growth Des.2003,3,791.(14)Ludwig,R.Angew.Chem.,Int.Ed.2001,40,1808.(15)The searching algorithm in the ConQuest Version1.9(CSD version5.28,May2007)was constrained to the presence of(i)at least onetetranuclear[(H2O)3(Cl)]–ring(i.e.,minimal cyclic fragment in our water-chloride network)with d(O···O))2.2–3.2Åand d(O···Cl) )2.6–3.6Å,and(ii)at least one crystallization water molecule andone chloride counter ion.All symmetry-related contacts were taken into consideration.(16)For2D networks with the[(H2O)3(Cl)]–core,see the CSD refcodes:AGETAH,AMIJAH,BEXVIJ,EXOWIX,FANJUA,GAFGIE, HIQCIT,LUNHUX,LUQCEF,PAYBEW,TESDEB,TXCDNA, WAQREL,WIXVUU,ZUHCOW.For3D network,see the CSD refcode:LUKZEW.(17)This analysis was run on1since we were unable to get1′in a sufficientamount due to the varying content of crystallization water in the samples obtained upon recrystallization of1.(18)(a)Das,S.;Bhardwaj,P.K.Cryst.Growth.Des.2006,6,187.(b)Wang,J.;Zheng,L.-L.;Li,C.-J.;Zheng,Y.-Z.;Tong,M.-L.Cryst.Growth.Des.2006,6,357.(c)Ghosh,S.K.;Ribas,J.;El Fallah, M.S.;Bharadwaj,P.K.Inorg.Chem.2005,44,3856.(19)A temperature below305°C has been used to avoid the eventualdecomposition of the compound upon rather prolonged heating.CG7010315Communications Crystal Growth&Design,Vol.8,No.3,2008785。
Characterization of Radar Backscatter翻译要点
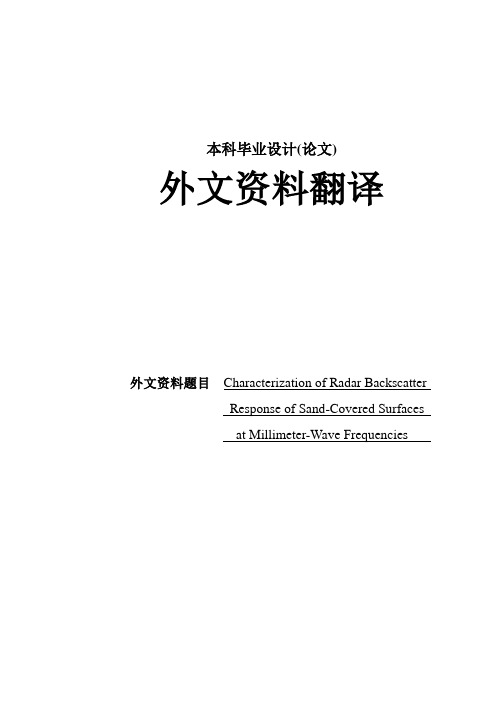
本科毕业设计(论文)外文资料翻译外文资料题目Characterization of Radar BackscatterResponse of Sand-Covered Surfacesat Millimeter-Wave Frequencies摘要摘要由于干沙的介电常数低和沙表面比较粗糙,沙漠的雷达成像会遭受不足的雷达回波低频微波信号。
然而,操作在毫米波段频率,用以矫正这一缺陷的重要雷达回波产生的表面和体积散射。
由于这样的事实,即砂表面粗糙度大,信号穿透到干砂,这是一个空气和砂颗粒尺寸与波长的一小部分的均匀混合物,产生相当大的体积散射。
本文探讨在干砂表面的面积和体积的散射,以奇特的砂表面的物理性能,发现在沙地沙丘覆盖的地区。
提出了一种非相干模型,描述了角依赖体积散射从干砂在存在一维起涟漪的空气/砂表面。
一组室内实验进行平滑和一维起涟漪的砂表面在ka波段证实,大量散射是毫米波频率,该模型正确地捕捉观察角关系当一维表面波纹出现。
关键词:毫米波(MMW)测量雷达体积散射摘要摘要目录i目录一、引言 (1)二、干砂的物理性质的表征 (5)三、干沙丘表面的造型雷达后向散射响应 (9)四、实验表征 (17)五、结论 (23)附录 (25)参考文献 (29)ii目录一、引言1一、引言世界各地的石油和天然气领域的探索,包括地区的特点是干旱或沙漠地区地震试验中经常使用的。
在这些地区,干沙层往往覆盖底层的基岩,并且其厚度变化从一个区域到下一个区域。
在沙层厚度的基石是低的沙区的地震试验通常是成功的。
如果砂层高于基岩的厚度是先验已知的,那么这些测试的成本可以显著降低。
双频干涉合成孔径雷达(SAR)测量(InSAR)已被提议作为一种映射沙层以上的基岩大面积的沙漠和干旱地区的厚度[1][2]的手段。
双频干涉合成孔径雷达的提议包含两个系统:高频和低频InSAR系统,两者都安装在一个平台上。
高频InSAR 技术被用于确定空气/砂界面的高度,而低频InSAR技术被用于确定的砂/基岩界面的高度。
电泳迁移率英文

电泳迁移率英文Electrophoretic MobilityElectrophoretic mobility is a fundamental concept in the field of electrophoresis, a widely used analytical technique in various scientific disciplines. This parameter describes the movement of charged particles or molecules under the influence of an applied electric field. Understanding and accurately determining electrophoretic mobility is crucial for the successful separation, identification, and characterization of biomolecules, such as proteins, nucleic acids, and other charged species.Electrophoresis is a powerful analytical tool that exploits the differential migration of charged particles or molecules in an electric field. When a charged particle or molecule is placed in an electric field, it will experience a force that causes it to move towards the electrode of opposite charge. The velocity at which the particle or molecule moves is directly proportional to the strength of the electric field and the electrophoretic mobility of the species.Electrophoretic mobility is a measure of the ease with which a charged particle or molecule moves through a medium under theinfluence of an electric field. It is typically expressed as the ratio of the particle's or molecule's velocity to the strength of the applied electric field. The unit of electrophoretic mobility is typically square centimeters per volt-second (cm²/V·s) or square meters per volt-second (m²/V·s).The electrophoretic mobility of a charged particle or molecule is determined by several factors, including the size, shape, and charge of the particle or molecule, as well as the properties of the surrounding medium, such as pH, ionic strength, and viscosity. Larger particles or molecules with a higher charge-to-size ratio generally exhibit higher electrophoretic mobilities, as they experience a greater force in the electric field.The determination of electrophoretic mobility is essential in various applications, such as:1. Protein and Nucleic Acid Analysis: Electrophoretic mobility is used to separate and characterize proteins and nucleic acids, such as DNA and RNA, based on their size, charge, and conformation.2. Cell and Organelle Separation: Electrophoretic mobility is employed to separate and purify different cell types, organelles, and subcellular components, such as mitochondria and nuclei.3. Colloidal and Nanoparticle Characterization: Electrophoretic mobility is used to study the surface charge and stability of colloidal systems and nanoparticles, which is crucial for their applications in fields like materials science, drug delivery, and environmental remediation.4. Enzyme and Antibody Analysis: Electrophoretic mobility is utilized to analyze the charge and purity of enzymes and antibodies, which is important for their characterization and downstream applications.5. Forensic and Clinical Diagnostics: Electrophoretic mobility is employed in various forensic and clinical diagnostic techniques, such as DNA profiling and the separation and identification of blood components.To determine the electrophoretic mobility of a charged particle or molecule, various experimental techniques can be employed, including capillary electrophoresis, gel electrophoresis, and microfluidic devices. These techniques often involve the application of an electric field and the measurement of the migration velocity of the particles or molecules.In conclusion, electrophoretic mobility is a fundamental concept in the field of electrophoresis, with numerous applications in various scientific and technological domains. Understanding and accuratelydetermining this parameter is crucial for the separation, characterization, and analysis of a wide range of charged species, from biomolecules to colloidal systems and nanoparticles.。
Advanced Materials Characterization
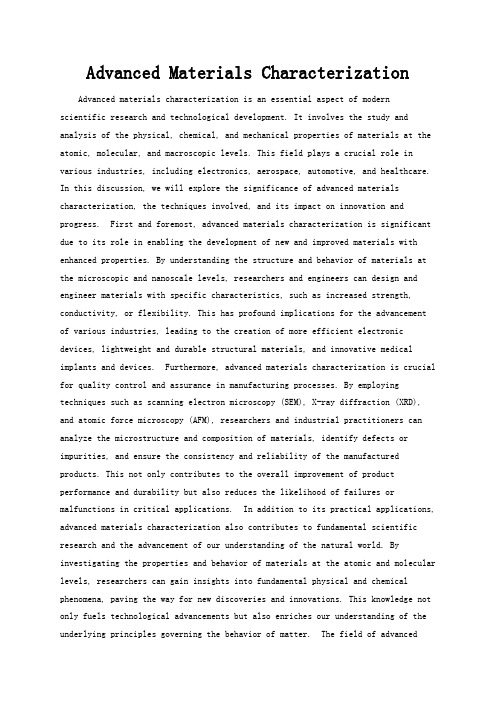
Advanced Materials CharacterizationAdvanced materials characterization is an essential aspect of modernscientific research and technological development. It involves the study and analysis of the physical, chemical, and mechanical properties of materials at the atomic, molecular, and macroscopic levels. This field plays a crucial role in various industries, including electronics, aerospace, automotive, and healthcare. In this discussion, we will explore the significance of advanced materials characterization, the techniques involved, and its impact on innovation and progress. First and foremost, advanced materials characterization is significant due to its role in enabling the development of new and improved materials with enhanced properties. By understanding the structure and behavior of materials at the microscopic and nanoscale levels, researchers and engineers can design and engineer materials with specific characteristics, such as increased strength, conductivity, or flexibility. This has profound implications for the advancement of various industries, leading to the creation of more efficient electronic devices, lightweight and durable structural materials, and innovative medical implants and devices. Furthermore, advanced materials characterization is crucial for quality control and assurance in manufacturing processes. By employing techniques such as scanning electron microscopy (SEM), X-ray diffraction (XRD), and atomic force microscopy (AFM), researchers and industrial practitioners can analyze the microstructure and composition of materials, identify defects or impurities, and ensure the consistency and reliability of the manufactured products. This not only contributes to the overall improvement of product performance and durability but also reduces the likelihood of failures or malfunctions in critical applications. In addition to its practical applications, advanced materials characterization also contributes to fundamental scientific research and the advancement of our understanding of the natural world. By investigating the properties and behavior of materials at the atomic and molecular levels, researchers can gain insights into fundamental physical and chemical phenomena, paving the way for new discoveries and innovations. This knowledge not only fuels technological advancements but also enriches our understanding of the underlying principles governing the behavior of matter. The field of advancedmaterials characterization encompasses a wide range of techniques and methodologies, each offering unique insights into the properties and behavior of materials. For instance, spectroscopic techniques such as Raman spectroscopy and infrared spectroscopy enable the analysis of molecular vibrations and chemical bonding in materials, providing valuable information about their composition and structure. On the other hand, imaging techniques like transmission electron microscopy (TEM) and atomic force microscopy (AFM) allow researchers to visualize and characterize materials at the nanoscale, offering unprecedented detail and resolution. Moreover, diffraction techniques such as X-ray diffraction (XRD) and neutron diffraction provide information about the crystal structure and phase composition of materials, aiding in the identification of crystalline phases and the determination of crystallographic parameters. Thermal analysis techniques, including differential scanning calorimetry (DSC) and thermogravimetric analysis (TGA), offer insights into the thermal stability, phase transitions, and decomposition behavior of materials, essential for understanding their thermal properties and behavior under varying conditions. In conclusion, advanced materials characterization plays a pivotal role in driving innovation, ensuring quality and reliability, and advancing our fundamental understanding of materials. By leveraging a diverse array of techniques and methodologies, researchers and engineers can gain profound insights into the properties and behavior of materials, leading to the development of new and improved materials with a wide range of applications. As technology continues to advance, the field of advanced materials characterization will undoubtedly remain a cornerstone of scientific and technological progress, shaping the future of various industries and contributing to the betterment of society as a whole.。
生物催化法制备低聚半乳糖的研究进展
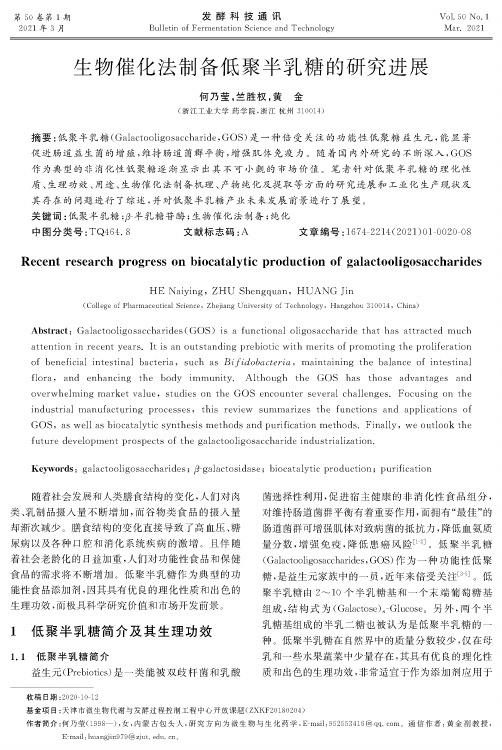
发酵科技通讯Bulletin of Fermentation Science and Technology Vol.50No.1 Mar2021第50卷第1期2021年3月生物催化法制备低聚半乳糖的研究进展何乃莹,竺胜权,黄金(浙江工业大学药学院,浙江杭州310014)摘要:低聚半乳糖(Galactooligosaccharide,GOS)是一种倍受关注的功能性低聚糖益生元,能显著促进肠道益生菌的增殖,维持肠道菌群平衡,增强肌体免疫力。
随着国内外研究的不断深入,GOS 作为典型的非消化性低聚糖逐渐显示出其不可小觑的市场价值。
笔者针对低聚半乳糖的理化性质、生理功效、用途、生物催化法制备机理、产物纯化及提取等方面的研究进展和工业化生产现状及其存在的问题进行了综述,并对低聚半乳糖产业未来发展前景进行了展望。
关键词:低聚半乳糖;0-半乳糖苷酶;生物催化法制备;纯化中图分类号:TQ464.8文献标志码:A文章编号:1674-2214(2021)01-0020-08 Recent research progress on biocatalytic production of galactooligosaccharidesHE Naiying,ZHU Shengquan,HUANG Jin(College of Pharmaceutical Science,Zhejiang University of Technology,Hangzhou310014,China)Abstract:Galactooligosaccharides(GOS)is a functional oligosaccharide that has attracted mucha t entioninrecentyears Itisanoutstandingprebioticwithmeritsofpromotingtheproliferationof beneficial intestinal bacteria,such as Bifidobacteria,maintaining the balance of intestinal flora,and enhancing the body immunity Although the GOS has those advantages and overwhelming marketvalue,studies on the GOS encounter several cha l enges Focusingonthe industrial manufacturing processes,this review summarizes the functions and applications of GOS,aswe l asbiocatalyticsynthesismethodsandpurificationmethods Fina l y,weoutlookthe futuredevelopmentprospectsofthegalactooligosaccharideindustrializationKeywords:galactooligosaccharides;0-galactosidase;biocatalytic production;purification随着社会发展和人类膳食结构的变化,人们对肉类、乳制品摄入量不断增加,而谷物类食品的摄入量却渐次减少。
Materials Characterization

Materials Characterization Materials characterization is a crucial aspect of scientific research and industrial development. It involves the analysis and understanding of the physical, chemical, mechanical, and structural properties of materials at various scales. This process is essential for ensuring the quality, performance, and reliabilityof materials in a wide range of applications, including manufacturing, construction, healthcare, and environmental protection. In this article, we will explore the significance of materials characterization from different perspectives, including its scientific, technological, and societal implications. From a scientific standpoint, materials characterization plays a fundamental role in advancing our understanding of the natural world and enabling the development of new technologies. By studying the properties and behavior of different materials, scientists can gain insights into their atomic and molecular structures, as wellas their interactions with external forces and environments. This knowledge forms the basis for the design and engineering of innovative materials with tailored properties, such as enhanced strength, conductivity, or biocompatibility. Moreover, materials characterization techniques, such as microscopy, spectroscopy, and diffraction, provide valuable data for theoretical modeling and simulation, allowing researchers to validate and refine their theoretical predictions. In the realm of technology and engineering, materials characterization is indispensablefor ensuring the performance, safety, and durability of various products and systems. For instance, in the aerospace industry, the characterization of composite materials used in aircraft structures is critical for assessing their resistance to fatigue, impact, and temperature variations. Similarly, in the field of electronics, the precise measurement of semiconductor properties is essentialfor optimizing the design and manufacturing of integrated circuits and electronic devices. Furthermore, in the medical field, the characterization of biomaterialsis essential for developing biocompatible implants, drug delivery systems, and tissue engineering scaffolds. Without accurate and comprehensive materials characterization, the development and optimization of these advanced technologies would be severely hindered. From a societal perspective, materialscharacterization has far-reaching implications for various aspects of everydaylife, including healthcare, energy, transportation, and environmental sustainability. For instance, in the healthcare sector, the characterization of pharmaceutical formulations and medical implants is crucial for ensuring their efficacy and safety. In the energy sector, the development of advanced materials for energy storage, conversion, and transmission relies heavily on accurate characterization data to optimize their performance and reliability. Moreover, in the context of environmental protection, the characterization of pollutants, waste materials, and renewable resources is essential for developing sustainable solutions for pollution control, waste management, and resource conservation. In conclusion, materials characterization is a multidisciplinary field with profound scientific, technological, and societal implications. Its significance extends across various industries and research domains, contributing to the advancement of knowledge, the development of innovative technologies, and the improvement of quality of life. As we continue to push the boundaries of materials science and engineering, the importance of robust and reliable characterization techniqueswill only grow, enabling us to unlock new possibilities and address complex challenges in the modern world.。
细菌脂多糖处理对斑马鱼免疫球蛋白亚型表达水平的影响

细菌脂多糖处理对斑马鱼免疫球蛋白亚型表达水平的影响王志平;胡庆玲;郝转【摘要】The healthy zebrafish were immersed in 20μg/mL LPS solution for 24 h ,and the expressions of IgM ,IgD and IgZ were analyzed by real‐time fluorescent quantitative PCR .In the control group ,the ex‐pression levels of IgZ and IgD were similar ,lower than that of IgM ,indicating a possible heighted role for the IgM isotype .After LPS challenge ,the expression of all Ig isotypes was significantly increased with a peak in 12 h .However ,the increase range of IgM and IgZ significantly exceeded that of IgD ,and their ex‐pression remained higher level than that in the control until24h ,indicating that IgM and IgZ played more important immunological role in response to antigen invasion .Moreover ,IgM up‐regulated its expression a bit more rapidly than IgZ did ,hinting at the clue that the humoral immune system might respond to LPS challenge a bit faster than the mucosal immune system .%以20μg/mL的细菌脂多糖溶液对斑马鱼进行浸泡处理24 h ,用实时荧光定量PCR技术分析斑马鱼IgM、IgD和IgZ基因表达水平的变化。
Materials Characterization
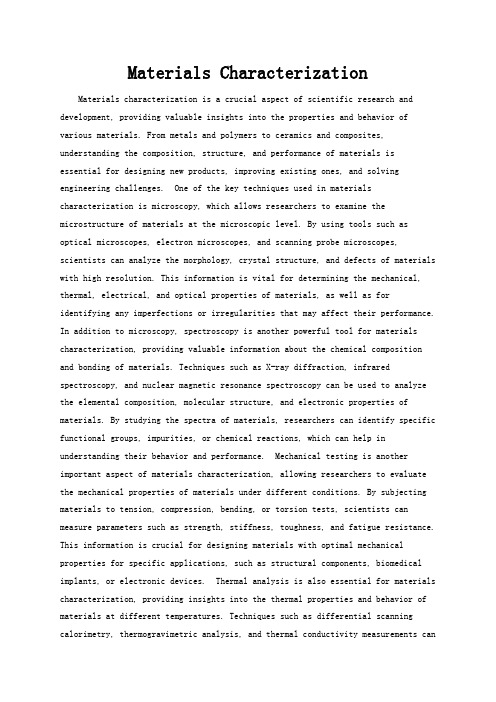
Materials Characterization Materials characterization is a crucial aspect of scientific research and development, providing valuable insights into the properties and behavior of various materials. From metals and polymers to ceramics and composites, understanding the composition, structure, and performance of materials isessential for designing new products, improving existing ones, and solving engineering challenges. One of the key techniques used in materials characterization is microscopy, which allows researchers to examine the microstructure of materials at the microscopic level. By using tools such asoptical microscopes, electron microscopes, and scanning probe microscopes, scientists can analyze the morphology, crystal structure, and defects of materials with high resolution. This information is vital for determining the mechanical, thermal, electrical, and optical properties of materials, as well as foridentifying any imperfections or irregularities that may affect their performance. In addition to microscopy, spectroscopy is another powerful tool for materials characterization, providing valuable information about the chemical compositionand bonding of materials. Techniques such as X-ray diffraction, infrared spectroscopy, and nuclear magnetic resonance spectroscopy can be used to analyze the elemental composition, molecular structure, and electronic properties of materials. By studying the spectra of materials, researchers can identify specific functional groups, impurities, or chemical reactions, which can help in understanding their behavior and performance. Mechanical testing is another important aspect of materials characterization, allowing researchers to evaluate the mechanical properties of materials under different conditions. By subjecting materials to tension, compression, bending, or torsion tests, scientists can measure parameters such as strength, stiffness, toughness, and fatigue resistance. This information is crucial for designing materials with optimal mechanical properties for specific applications, such as structural components, biomedical implants, or electronic devices. Thermal analysis is also essential for materials characterization, providing insights into the thermal properties and behavior of materials at different temperatures. Techniques such as differential scanning calorimetry, thermogravimetric analysis, and thermal conductivity measurements canbe used to study the phase transitions, thermal stability, and heat transfer properties of materials. This information is critical for designing materials that can withstand high temperatures, resist thermal degradation, or conduct heat efficiently, depending on the application requirements. Electrical and magnetic characterization is another important aspect of materials research, particularlyfor electronic and magnetic materials. By measuring parameters such as resistivity, conductivity, dielectric constant, and magnetic susceptibility, researchers can evaluate the electrical and magnetic properties of materials. This information is essential for designing electronic devices, sensors, and magnetic storage media,as well as for understanding the behavior of materials in electromagnetic fields. Overall, materials characterization plays a crucial role in advancing scientific knowledge, technological innovation, and industrial development. By employing a combination of microscopy, spectroscopy, mechanical testing, thermal analysis, and electrical characterization techniques, researchers can gain a comprehensive understanding of the properties and behavior of materials, enabling them to design new materials with tailored properties and improved performance. This interdisciplinary approach to materials characterization is essential for addressing complex engineering challenges, developing sustainable materials, and advancing various fields of science and technology.。
Materials Characterization

Materials Characterization Materials characterization is a crucial aspect of materials science and engineering, playing a significant role in understanding the properties and behavior of various materials. It involves the use of different techniques and methods to analyze the structure, composition, and properties of materials at the micro- and nano-scale. This process is essential for the development and improvement of materials for various applications, including in the fields of manufacturing, construction, electronics, and healthcare. One of the primary reasons why materials characterization is important is that it provides valuable insights into the structure-property relationships of materials. By understanding the internal structure of a material and how it influences its properties, scientists and engineers can make informed decisions about the selection and design of materials for specific applications. For example, in the aerospace industry, materials characterization is used to ensure that the materials used in aircraft components have the necessary strength, durability, and heat resistance to withstand the harsh conditions of flight. Moreover, materials characterization is essential for quality control and assurance in manufacturing processes. By analyzing the composition and microstructure of materials, manufacturers can identify any defects or inconsistencies that may affect the performance and reliability of the final product. This is particularly critical in industries such as automotive manufacturing, where the use of high-performance materials is essential for ensuring the safety and longevity of vehicles. In addition, materials characterization plays a crucial role in the development of new and advanced materials with enhanced properties. By studying the structure and behavior of materials at the atomic and molecular levels, researchers can identify opportunities for improving their performance, such as increasing strength, conductivity, or corrosion resistance. This has led to the development of innovative materials such as carbon nanotubes, graphene, and shape memory alloys, which have found applications in various industries. Furthermore, materials characterization is essential for understanding the degradation and failure mechanisms of materials over time. By studying how materials respond to environmental factors, such as temperature, humidity, and mechanical stress,researchers can develop strategies to mitigate degradation and improve thelifespan of materials. This is critical for infrastructure and construction materials, where durability and longevity are paramount for ensuring the safety and stability of buildings and bridges. Another important aspect of materials characterization is its role in advancing scientific knowledge and understanding of the natural world. By studying the properties and behavior of materials, scientists can gain insights into fundamental physical and chemical phenomena, leading to new discoveries and innovations. This has led to the development of new theories and models that have broadened our understanding of the natural world and paved the way for technological advancements. In conclusion, materials characterization is a fundamental aspect of materials science and engineering, with far-reaching implications for various industries and scientific disciplines. Its importance lies in its ability to provide insights into the structure-property relationships of materials, ensure quality control in manufacturing processes, facilitate the development of new materials, and advance scientific knowledge. As technology continues to advance, the role of materials characterization will only become more critical in shaping the future of materials and their applications in the modern world.。
谐振子密度偏差引起的频率裂解的分析

谐振子密度偏差引起的频率裂解的分析任顺清;赵洪波【摘要】In order to study the frequency cracking caused by the nonuniform of density distribution,the dynamics equations of resonator under the nonuniform of density distribution were established by way of Bubonov—Galerkin method which is common used for solution of differential equations,the state equation was established by the dynamics equations,and then the system characteristic equations werederived.According to the system characteristic equations,two second order natural frequency caused by the density nonuniformity around the hemispherical of vibration system and the expression of frequency cracking were solved.%为研究半球谐振子密度不均匀引起的频率裂解,首先利用解微分方程的布勃诺夫-加廖尔金法建立了谐振子环向密度分布不均匀的动力学方程,根据动力学方程建立了振动系统的状态方程,进而推导了系统的特征方程,根据特征方程解出了在谐振子存在环向密度不均匀的前提下,振动系统存在的两个二阶固有频率,最后求解了固有频率裂解的表达式.【期刊名称】《哈尔滨工业大学学报》【年(卷),期】2012(044)003【总页数】4页(P13-16)【关键词】半球谐振子;密度分布不均匀;频率裂解【作者】任顺清;赵洪波【作者单位】哈尔滨工业大学空间控制与惯性技术研究中心,哈尔滨150001;哈尔滨工业大学空间控制与惯性技术研究中心,哈尔滨150001【正文语种】中文【中图分类】U666.1当半球谐振子的密度、厚度、品质因数等参数分布不均匀,并存在沿半球谐振子周向的四次谐波时,谐振子的二阶振型将出现两个相互间展成45°的固有轴,沿这两个不同固有轴的二阶弯曲振型对应的固有频率分别达到极大和极小值.两个固有频率差称作频率裂解.文献[1]只给出了频率裂解的公式,并没有给出详细的推导过程.如果对谐振子的激励不沿固有轴方向,频率裂解会使谐振子振型的驻波向固有轴缓慢漂移直至振动沿固有轴方向,从而导致陀螺漂移.本文将针对密度分布不均匀引起的频率裂解进行详细推导,得出与文献[1]不同的更加精确的频率裂解表达式.如图1所示,半球壳谐振子坐标系为OXYZ,半球壳谐振子中曲面一点的矢径为R,把经线和纬线作为坐标曲线α、β,它们的切线单位矢量e1、e2和法线单位矢量e3组成1个右手局部坐标系.假设半球壳的中曲面任一点在局部坐标系的位移为M=ue1+ve2+we3.将谐振子各点的位移按不可拉伸薄壳的二阶固有振型展开得式中:U(α)=V(α)=sinαtan2(α/2),W(α)=-(2+cosα)tan2(α/2)为二阶固有振型的瑞利函数,p(t)、q(t)为按二阶固有频率振动的位移函数.由于密度不均匀,将密度ρ沿谐振子周向角β展开成Fourier级数的形式,如下: 式中ai为密度展开式第i次谐波余弦幅值,bi为密度展开式第i次谐波正弦幅值. 根据文献[2],在有速率Ω和角加速度的情况下,将密度展开式代入谐振子的惯性力,然后考虑阻尼和位置激励力F,并利用布勃诺夫-加廖尔金法重新计算并化简,忽略详细的推导过程得到半球谐振子二阶固有振型的动力学方程如下:式中:阻尼l=c0/Q,Q为半球谐振子的品质因数;E为杨氏模量;h为半球谐振子薄壳的厚度;γ为半球谐振子材料的泊松比;ω0为不考虑频率裂解时的半球谐振子二阶固有频率;W'、W″、W‴、W(4)表示瑞利函数W对周向角.β求一、二、三、四阶导数,其他类同.下面将证明对于动力学方程(1),有两个二阶固有频率ω1、ω2的存在,本节将推导出固有频率裂解表达式.设x=,y=,则由式(1)得根据以上各式可以建立以振动位移和速度的状态方程为矩阵B的特征方程为对式(3)进行处理,令λ=s-a33/4得设特征多项式(4)的4个根为本文第3节将要证明当阻尼l较小时有将 s1、s2、s3、s4 代入如下方程:并与式(4)比较得利用韦达定理解一元二次方程并假设ω1>ω2得对式(1)中的各参数如m0、m1等进行积分计算,并带入式(2)中B矩阵的各个参数,再计算a00、a22,最后对上式化简得由于ε4≪ ρ0,所以其中为四次谐波的绝对值幅值,ε'4=ε4/ρ0为密度偏差四次谐波的相对值.公式(5)是在阻尼l较小的假设下推导出来的,阻尼l由品质因数Q决定,因此下面将利用实际数值讨论品质因数Q对公式(6)的影响.目前国内生产谐振子的材料为熔融石英,其密度ρ=2 200 kg·m-3,杨氏模量E=7.67×1010N·m-2,泊松比γ =0.17,中曲面半径R=0.015 m,厚度h=0.85×10-3m,根据文献[2]计算得ω0=18 727 rad·s-1,现对 a4b4取不同的值进行验证,结果如表1所示.国内某研究所生产的半球谐振子品质因数Q能达到107,因此根据本文的假设推导的公式(5)是正确的.1)在半球谐振子环向密度不均匀的情况下建立了谐振子的动力学方程;2)根据动力学方程建立了振动位移、速度的状态方程,根据系统的特征方程证明了谐振子在密度不均匀存在四次谐波的情况下,存在两个二阶固有频率;3)在忽略了谐振子阻尼的情况下,简化了频率裂解的解析表达式.上面的方法在分析谐振子其他缺陷比如厚度、密度、杨氏模量、品质因数不均匀时的频率裂解提供了一种分析方法.【相关文献】[1]МАТВЕЕВ В А,ЛИПАТНИКОВ В И,АЛЕИН А В.Проектирование. Волновоготвердотелъного гироскопа[M]. Москва:Издателъство МГТУ имениН.Э.Баумана,1998:1 -43.[2]赵洪波,任顺清,李巍.半球谐振子动力学方程的建立及固有频率的计算[J].哈尔滨工业大学学报,2010,42(11):1702 -1706.[3]EMILY L B,ALLAN Y L.In-flight characterization of cassini inertial reference units[C]//AIAA Guidance,Navigation and Control Conference and Exhibit.Hilton Head,South Carolina:AIAA,2007 -6340.[4]JIAYI Q,XUE C,SHUNQING R,et al.Dynamic error mechanism analysis of HRG[C]//ISSCAA2008,Shenzhen:[s.n.],2008:12.[5]ZHBANOV Y K.Vibration of a hemispherical shell gyro excited by an electrostaticfield[J].Mechanics of Solids,2008,43(3):328 -332.[6]ZHBANOV Y K,ZHURAVLEV V P.Effect of movability of the resonator center on the operation of a hemispherical resonator gyro[J].Mechanics of Solids,2007,44(3):851-859.[7]FAN Shang-chun,LIU Guang-yu,WANG Zhen-jun.On flexural vibration of hemispherical shell[J].Applied Mathematics and Mechanics,1991,12(10):1023 -1030.。
- 1、下载文档前请自行甄别文档内容的完整性,平台不提供额外的编辑、内容补充、找答案等附加服务。
- 2、"仅部分预览"的文档,不可在线预览部分如存在完整性等问题,可反馈申请退款(可完整预览的文档不适用该条件!)。
- 3、如文档侵犯您的权益,请联系客服反馈,我们会尽快为您处理(人工客服工作时间:9:00-18:30)。
Characterization of a Differential Radio-Frequency Single-Electron TransistorJ.F. Schneiderman*, P. Delsing†,‡, M.D. Shaw*, H.M. Bozler*, and P.M.Echternach‡‡Jet Propulsion Laboratory, California Institute of Technology, Pasadena, CA 91109, USA *University of Southern California, Dept of Physics and Astronomy, Los Angeles, CA 90089-0484, USA †Chalmers University of Technology, Microtechnology and Nanoscience, MC2, 412 96 Göteborg, SwedenAbstract. We have fabricated and characterized a new type of electrometer that couples two parallel single-electron transistors (SETs) to a radio-frequency tank circuit for use as a differential RF-SET. We demonstrate operation of this device in summing, differential, and single-SET operation modes, and use it to measure a Coulomb staircase from a differential single Cooper-pair box. In differential mode, the device is sensitive to uncorrelated input signals while screening out correlated ones.Keywords: RF-SET, Coulomb blockade, 1/f noise, offset charge, SCB.PACS:03.67.Lz, 73.23.Hk, 74.50.+r, 85.25.Cp, 85.35.Gv.The single-electron transistor1,2(SET) is widely used as an extremely sensitive electrometer, having found a niche in a number of applications involving ultra-sensitive measurements. Unfortunately, background charge fluctuations3 have been a problem plaguing single electron devices since their inception. Such charges are thought to be a primary cause of the 1/f noise that limits the resolution of precision charge measurements. Over time, a number of important technological improvements have been made to the SET, such as radio-frequency operation4(RF-SET). The RF-SET is operated at sufficiently high frequencies for the 1/f noise level to be substantially lowered.In many applications, it is desirable to measure the difference in charge between two parts of the same object. For example, Buehler et al. recently used two independent RF-SETs to measure the motion of charge between two phosphorous dots embedded in silicon5. For this measurement, independent resonant circuits were used for each SET, and the readout signal was subtracted after RF detection. Furthermore, one proposed variety of superconducting charge qubit is the differential single Cooper-pair box (DSCB)6,7, which requires measuring the difference in charge between two islands separated by a Josephson junction.In this letter, we report on the experimental demonstration of a differential RF-SET (DRFSET) consisting of two parallel SETs acting as the dissipative element of a single resonant LC circuit (see Figure 1). Each SET has a separate transfer function which reflects the change in its conductance as a voltage is applied to its respective gate. To achieve differential readout, the SETs are biased so that their responses are of opposite sign. In this mode, when a fluctuation in charge couples to both SET islands, the conductance change of one island will be opposite in sign to that of the other, leading to a cancellation of the change in the conductance of the parallel SETs. In other words, the DRFSET is insensitive to collective fluctuations in charge affecting both SET islands together. This mode of operation also allows for a strong differential readout since increasing the charge coupled to one SET while decreasing the charge coupled to the other will produce a correlated and reinforced change in conductance of the parallel SETs, and hence a large change in the dissipation of the LC tank circuit for readout.Other modes of DRFSET operation are also possible, depending on where each SET is biased on its transfer function (see Figure 2). The simplest mode of operation is to bias one SET at an insensitive part of its transfer function and the other at its highest sensitivity. This “single-SET” mode is useful for characterization of the separate SETs. Finally, one can operate the DRFSET in a “common” or summing mode which is closer to the usual RF-SET setup.Summing mode is made possible by biasing each SET island so that the signs of their respective responses are the same. Both SETs will then respond in the same way to an overall change in charge on theirrespective islands.FIGURE 1.Circuit diagram showing the DSCB with DRFSET readout. C SG1, C SG2, C QG1, C QG2, C C1, and C C2 are the SET gate, qubit gate,and coupling capacitances for SET1 and SET2, respectively.L and C are the inductance and capacitance of the tank circuit. Inset: SEM image of the pictured circuit. The red and yellow features are the two SETs, while the cyan and violet features form the DSCB.As mentioned above, an application of the DRFSET of particular interest for quantum computing is a charge readout for the differential single Cooper-pair box (DSCB). The DSCB qubit consists of two islands coupled by a small tunnel junction, with the relevant quantum states being the difference in charge between the two islands. Even though the potential of the islands may fluctuate with offset charges, the difference in charge between them should be relatively unaffected unless the fluctuating charge is very close to the DSCB itself. The DRFSET becomes an ideal readout device for the DSCB by coupling each of the DRFSET islands to a separate DSCB island.We have achieved operation of a differential RF-SET and used it as a differential electrometer to measure a Coulomb Staircase from a DSCB. The inset of Figure1 shows an SEM picture of a DSCB with its left and right islands coupled to separate islands of a DRFSET. The samples were fabricated using a standard double-angle shadow mask evaporation technique2. Each island of the DSCB has a gate electrode which is used to push and pull charges from one island to the other,while each SET has its own tuning gate used to bias it at different operating points. Operation in the RF mode is achieved by sending an RF signal to an LC tank circuit for which the parallel SETs act as the dissipative element4.We have measured the voltage of the reflected signal as a function of both gate voltages with the SETs biased at the double-Josephson-quasiparticle (dJQP) peak8,9. Figure2 shows the results of such ameasurement, and indicates gate voltage values appropriate for each mode of operation. Regions where the slope of the curve is large represent operating points of high SET sensitivity.At point a, for example, the reflected signal is essentially independent of the voltage on gate 1, but depends strongly on gate voltage 2, and thus represents a point where only SET2 is sensitive (single-SET mode). Similarly, at point b only SET1 is sensitive. At points c(summing mode) both SETs are sensitive and biased on the same slope, so an increase in both gate voltagesyields a net sum change in the reflected RF signal. FIGURE 2. Coefficient of reflected power as a function of both SET gate voltages. Four modes of operation are shown: a, single-SET mode, SET1 insensitive;b, single-SET mode,SET2 insensitive;c, summing mode; d, differential mode.Finally, at points d(differential mode), where the SETs are biased on opposite slopes, an increase in both gate voltages results in an opposing change in the conductance of each SET, tending to cancel out the change in reflected power from the overall device. However, a change in each gate voltage in opposite directions will yield a reinforced change in the overall reflected power. This makes the DRFSET an ideal device for reading out the charge state of a DSCB.To demonstrate operation of the DRFSET, we applied small amplitude (0.01e PP) low-frequency signals to each of the SET gates, and a third signal to both qubit gates. Each signal had a unique and coprime frequency to avoid self-mixing or mixing with harmonic and subharmonic modes.We applied9- and 11-Hz signals to SET1 and SET2respectively, and a 13-Hz “common-mode” signal to the gates of the DSCB,which couples to the SET gates with equal strength via the cross-capacitances. By varying the DC offset levels of the 9- and 11-Hz signals, we could vary the operating point of the DRFSET, changingbetween summing,differential, and single-SET modes. We examined the reflected signal from the DRFSET using a spectrum analyzer, as shown in Figure3.In Figures3a and 3b, the SETs are biased in single-SETmode as described above.FIGURE 3.DRFSET readout spectra. Graphs a-d correspond to the modes of operation described in Figure 2.Figure3c shows the operation of the DRFSET in the summing mode, while Figure3d shows results for operation in differential mode.Observe the peak corresponding to the13-Hz common mode signal is quite pronounced while in summing mode,whereas operation in the differential mode causes this signal to be suppressed below the1/f noise floor. This demonstrates that a DRFSET operated in differential mode is insensitive to a common mode signal, and thus has the advantage of being immune to correlated noise.In a similar fashion, we examined noise spectra at a variety of different SET operating points. At high frequencies (tens of kHz and above),the noise floor remained unchanged even when comparing areas of high SET sensitivity to areas of total insensitivity. This result can be explained by the assumption that the noise floor is dominated by amplifier noise above a few kHz. However, at frequencies below a few hundred Hz,noise levels followed the gain of the SETs, irrespective of readout mode. We ensured the DRFSET was insensitive to correlated input signals not only for the select frequency used for the “common mode” signal described above,but for all frequencies up to several hundred Hz.We applied a common white noise signal to both qubit gates and measured spectra in the differential and summing readout modes. The differential readout mode showed a reduction in the correlated noise by9.8±0.2 dB. The fact that the level of the1/f noise floor does not change between summing and differential modes implies there is no correlation in the noise felt by the two SETs. This in turn indicates that most of the 1/f noise results from charge fluctuators which are much closer to either SET than the distance between each SET (5 µm). This result is compatible with those of Zorin et al.3, which indicate a 15% correlation of noise for SETs separated by 0.2 µm.As a further demonstration of the DRFSET, we have measured a Coulomb staircase10from a differential single Cooper-pair box with the DRFSET. By applying a voltage ramp to one side of the DSCB and a similar ramp of opposite sign to the other, charge was transferred from one island of the DSCB to the other. This transfer of charge was then measured by the DRFSET operating in differential mode.In conclusion, we have experimentally demonstrated a differential radio-frequency single electron transistor in its four modes of operation. Differential readout was verified by measuring a Coulomb staircase from a differential single Cooper-pair box. At low frequencies, the 1/f noise floor was shown to be independent of the operating mode, implying a lack of1/f noise correlation between the two SET islands situated 5 µm apart.GWe thank Rich E. Muller for the electron-beam lithography.Research was performed at the Jet Propulsion Laboratory, California Institute of Technology,under a contract with the National Aeronautics and Space Administration. We acknowledge support by the National Security Agency and the Advanced Research and Development Activity.REFERENCES1. D.V. Averin and K.K.Likharev,J. Low Temp.Phys.62,345 (1986).2.T.A.Fulton and G.J. Dolan,Phys. Rev. Lett.59, 109(1987).3. A.B.Zorin, F.-J. Ahlers, J. Niemeyer, T. Weimann, H.Wolf, V. A. Krupenin, and S. V. Lotkhov,Phys. Rev. B53, 13682 (1996), and references therein.4.R.J. Schoelkopf, P. Wahlgren, A.A. Kozhevnikov, P.Delsing,and D.E. Prober,Science280, 1238 (1998).5.T. M. Buehler,D. J. Reilly, R.P. Starrett, A.D. Greentree,A.R. Hamilton,A.S. Dzurak, and R.G. Clark,Appl. Phys.Lett.86, 143117 (2005).6. A. Shnirman, G. Schon, and Z. Hermon, Phys. Rev.Lett.79, 2371 (1997).7.K. Bladh, D. Gunnarsson, G. Johannsson, A. Käck, G.Wendin, A. Aassime, M. Taslakov, and P. Delsing, Physica Scripta T102, 167 (2002).8.S.L. Pohlen, Ph.D.Thesis, Harvard University,Cambridge, MA. (1999).9. A.A. Clerk, S.M. Girvin, A.K. Nguyen,and A.D. Stone,Phys. Rev.Lett.89, 176804 (2002).10.I.O. Kulik and R.I. Shekter, Sov. Phys. JETP41, 308(1975).。