Integrated Energy Systems Cooling, Heating & Power Overview
基于随机优化的综合能源系统能量管理

第48卷第1期!"大学学% (自然科学版)Vol.48 No.l 2018 年1月JO U R N ALO F SO U TH E A STU N IVE R SITY(N a tu ra l S cien ce E d itio n)Jan. 2018D O I:10. 3969/j. i s s n.1001 -0505.2018. 01.008基于随机优化的综合能源系统能量管理季振亚12黄学良12张梓麒12孙厚涛12赵家庆3李军4(东南大学电气工程学院,南京210096)(2江苏省智能电网技术与装备重点实验室,南京210096)(3国网苏州供电公司,苏州215004)(4南京工程学院电力工程学院,南京211167)摘要:为提 能源系统经济性,并削减电动汽车广泛应用后增加的用电高峰负荷,提出了一 种包含随机优化与解算法的 能量管理策略.在对多能流子系统耦合与设备运行约束建模后,结合电动汽车有序充电策略,建立包含 滚动的随机规划下的能量管理模型.为了降能量管理在线运行的时间,一方面采用场景生成与削减技术实入 预测场景集的合理利用,另一方 解 用Benders分解算法实 .算例结 :所提方法与不考虑随机优化的方法 ,系统运行的总用能 显;与不考虑场景削减及Benders分解的随机优化方法 ,总用能 稍有提高,但运 间显著下降.关键词:综合能源系统;随机优化;电动汽车有序充电;Benders分解中图分类号:X703.5 文献标志码:A文章编号:1001 -0505(2018)01-0045-09Energy management for integrated energy systems based on stochastic optimization Ji Zhenya1’2Huang Xueliang1’2Zhang Ziqi1’2Sun Houtao1,2Zhao Jiaqing3Li Jun4(!S ch o o l of E le ctrica l E n gin eerin g,S o u th e a s t U n iversity,N a n jin g210096,C h in a)(2K ey L a b o ra to ry of J ia n g s u P ro v in ce S m a r t G rid T ech n o lo g y a n d E q u ip m en t,N a n jin g210096 ,C h in a)(3S ta te G rid S u zh o u P o w er S u p p ly C o m p a n y,S u zh o u215004 ,C h in a)(4S ch o o l of E lectrica l E n g in e e rin g,N a n jin g In s titu te of T ech n o lo g y,N a n jin g2 11167,C h in a)Abstract: To rise the economical efficiency of integrated energy system and reduce p e a e lectricity loads by the widespread u se of electric vehicles ,a new energy management strategy i s presentedbased on a stochastic optimization and a paallel solving algorithm to achieve fast solution. The receding horizon based energy management model in a stochastic programming after the formulation of a n integrated energy system with constraints , and i s implemented with a coordinating chaging strategy for electric vehicles. To reduce the time cost of online operation, a scenario generation and r eduction method i s used to apply suitable predicted scenario sets, and theBenders decomposition technique i s adopted to execute paallel computing. The simulation results show that the p roposed method achieves significantly decrease of the operation cost than those without stochastic optimization. Compared with a stochastic method for neither scenario reduction norBenders decomposition , computing time i s notably declined by l i t t l e operational cost to increase.Key words:integrated energy system;stochastic optimization;electric vehicle coordinated cha- ging; Benders decomposition综合能源系统(integrated energy system, IES) 高效和梯级利用,具有调整能源结构、促进节能减能流子系统相对割裂的状态,实现能排等社益,符合我国能源互联网发展需收稿日期:2017-07-15.作者简介:季振亚(198—),女,博士生;黄学良(联系人),男,博士,教授,博士生导师,xl h u a n g@ su e d u.c.基金项目:科技部重点基础研发计划资助项目(2016YFB0101800)、江苏省科技支撑计划资助项目(B E2014023)、国家电网公司总部科技资助项目(SG T Y H T/14-J S-188).引用本文:季振亚,黄学良,张梓麒,等.基于随机优化的综合能源系统能量管理[J].东南大学学报(自然科学版),2018,48(1) :5-53.D O I:10.3969/j.issn.1001-0505.2018.01.008.46东南大学学报(自然科学版)第48卷[1气I E S的能量管理充分考虑多能流耦合,如热电联产机组(combined heat and power,C H P)、电动热泵(electrical heat p u m p,E H P)等设备,在满子系统供需平运束的前提下,提高能源合率、降能成本、促进生能,升经济与环境效益来直接提高I E S的实价值[3+.其中,电动汽车的发展使电力系能源系统的耦合成为.然而,电动汽车充电的随机性使得规模化电动汽车接能带来电力系统新的峰[5].. 进‘加入有电策略,I E S能量管适长的电动汽车充电,减少承担高峰的系统建设.模型预测控制(model predictive control,M P C)于20世纪70年代的工业控制领域,在当前时刻预测模型求解预测的控制列,但仅执前时刻的控制,下刻重复[6].近年来,基于上述原理的能量管理关注[],于用能支出的[8].常见的M P C基于单一确定性模型,即D M P C(determined M P C). .生能源发电系统(renewable energy system,RES) 出力、负荷等短期预测能的>11],变机性逐精确的表,基于随机的模型预测控制(stochastic M P C,S M P C)日益重视.S M P C适用于型概率分布和具有特征的随机性模型[2],于变导致S M P C计算时间与空间成本极大增加,因率的场景表达随机性[13_14].文献[15 ]验虑随机的微网能管济性,但随机场景较少、电动汽车:恒定、未考虑滚动;文献[1]实现了风储系统基于滚动的随机,未涉及电动汽车,且预测场景有限;文献[7]考虑风机场景下的电动汽车有电,虑滚动计算度,且电动汽车变.总体来说,目前绝大虑随机性与滚动求解的能量管R E S为主,使考虑了电动汽车接入,也往往忽略电动汽车在I E S中流动的特征.实中,在M P C预测时域内,已接入IES 的电动汽车车主驶离导致该车辆,新抵达的电动汽车也有可能接入空闲的充电粧,该特征其他固定型设备特征产生.而当前的电动汽车有电策略仅针对单一车辆本身,对I E S中接入电动汽车的变虑.此外,目前I E S能量管仅考虑单一的解策略,即在包含随机性与加解速度中从简,但M P C方法包括滚动的特征,对实解能力要求较高,上述简生果受削计算速度受制约的问题.本文以I E S包含的电动汽车充电粧为有序充电的制单,电为,S M P C策略,建立I E S总用能成本最低的2阶段随机规划模型,解期决策变量,其中,考虑变量的随机性.为实现滚动求解,一方场景生削减技术实现对变预测场景集的合,另一方合B e d e s分解算法进高计算速度.所提方法个区I S中验证,结果表明该方法在保证求解速度的基础上,具有良好的经济性.1区域多能流综合能源系统建模1.1系统结构模型I E S由分布于的电力子系统、天然气子系统、热力子系统、交通子系统等组成,图1 .耦合实现子系统间的耦合,消耗系中能生其能,括C H P、E H P、燃气锅炉、R E S等.非耦合针对单一能源的生产、使储,包括、储电单元、储热单元等.在能量管理系统的综合调度下,IES 为整协益.图1多能流区域综合能源系统结构示意图1.2多能流耦合约束I E S能量管理的先决 是保持供需平衡.定模型,合 矩阵%= |%,2,…,合 矩阵M nc =IM1M2,…,其中分别为耦合元件http://journal,.cn第1期季振亚,等:基于随机优化的综合能源系统能量管理47与非親合元件数;输出端为负荷矩阵i d = U i,L2,R E S以风机为例,约束条件为…,4丨T,为负荷种类;耦合元件在各能流间作用 关系通过親合矩阵C建立,矩阵元素^#0 表示负荷《与親合元件X之间存在转换关系,否则 无关.约束关系为L d =--CWc+Mn3L13434a^1a^13^1343434313%.图1中,输出端包括用电功率与热功率,电动汽车充电负荷功率包含于内,B P ',=、,,+ ,、,,为一般用电负荷功率,功率平衡表达式为-P,P e,[n A f0P-P,_i_■Pe x,f+Pb/ P,■nc,f n0 n h■Yr P…,■_⑵式中,,',分别为f时刻c h p电转换效率和热 转换效率;毛,为二元变量,X。
外显、灵活、多维、高效——从2022中国国际太阳能十项全能竞赛看建筑与光伏一体化设计趋势
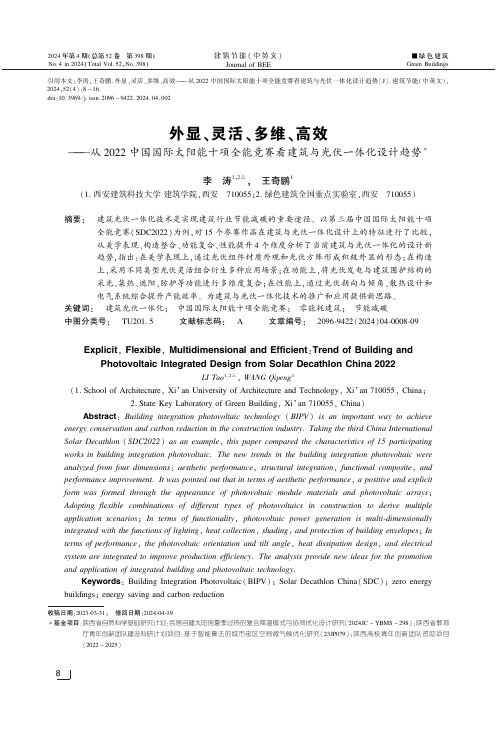
2024年第4期(总第52卷㊀第398期)No.4in2024(TotalVol.52ꎬNo.398)建筑节能(中英文)JournalofBEEʏ绿色建筑GreenBuildings引用本文:李涛ꎬ王奇鹏.外显㊁灵活㊁多维㊁高效 从2022中国国际太阳能十项全能竞赛看建筑与光伏一体化设计趋势[J].建筑节能(中英文)ꎬ2024ꎬ52(4):8-16.doi:10.3969/j.issn.2096-9422.2024.04.002收稿日期:2023 ̄03 ̄31ꎻ㊀修回日期:2024 ̄04 ̄19∗基金项目:陕西省自然科学基础研究计划:民居自建太阳房夏季过热的复合降温模式与协同优化设计研究(2024JC-YBMS-298)ꎻ陕西省教育厅青年创新团队建设科研计划项目:基于智能算法的城市街区空间微气候优化研究(23JP079)ꎻ陕西高校青年创新团队资助项目(2022-2025)外显㊁灵活㊁多维㊁高效从2022中国国际太阳能十项全能竞赛看建筑与光伏一体化设计趋势∗李㊀涛1ꎬ2әꎬ㊀王奇鹏1(1.西安建筑科技大学建筑学院ꎬ西安㊀710055ꎻ2.绿色建筑全国重点实验室ꎬ西安㊀710055)摘要:㊀建筑光伏一体化技术是实现建筑行业节能减碳的重要途径ꎮ以第三届中国国际太阳能十项全能竞赛(SDC2022)为例ꎬ对15个参赛作品在建筑与光伏一体化设计上的特征进行了比较ꎬ从美学表现㊁构造整合㊁功能复合㊁性能提升4个维度分析了当前建筑与光伏一体化的设计新趋势ꎬ指出:在美学表现上ꎬ通过光伏组件材质外观和光伏方阵形成积极外显的形态ꎻ在构造上ꎬ采用不同类型光伏灵活组合衍生多种应用场景ꎻ在功能上ꎬ将光伏发电与建筑围护结构的采光㊁集热㊁遮阳㊁防护等功能进行多维度复合ꎻ在性能上ꎬ通过光伏朝向与倾角㊁散热设计和电气系统综合提升产能效率ꎮ为建筑与光伏一体化技术的推广和应用提供新思路ꎮ关键词:㊀建筑光伏一体化ꎻ㊀中国国际太阳能十项全能竞赛ꎻ㊀零能耗建筑ꎻ㊀节能减碳中图分类号:㊀TU201 5㊀㊀㊀文献标志码:㊀A㊀㊀㊀文章编号:㊀2096 ̄9422(2024)04 ̄0008 ̄09ExplicitꎬFlexibleꎬMultidimensionalandEfficient:TrendofBuildingandPhotovoltaicIntegratedDesignfromSolarDecathlonChina2022LITao1ꎬ2әꎬWANGQipeng1(1.SchoolofArchitectureꎬXi anUniversityofArchitectureandTechnologyꎬXi an710055ꎬChinaꎻ2.StateKeyLaboratoryofGreenBuildingꎬXi an710055ꎬChina)㊀㊀Abstract:Buildingintegrationphotovoltaictechnology(BIPV)isanimportantwaytoachieveenergyconservationandcarbonreductionintheconstructionindustry.TakingthethirdChinaInternationalSolarDecathlon(SDC2022)asanexampleꎬthispapercomparedthecharacteristicsof15participatingworksinbuildingintegrationphotovoltaic.Thenewtrendsinthebuildingintegrationphotovoltaicwereanalyzedfromfourdimensions:aestheticperformanceꎬstructuralintegrationꎬfunctionalcompositeꎬandperformanceimprovement.ItwaspointedoutthatintermsofaestheticperformanceꎬapositiveandexplicitformwasformedthroughtheappearanceofphotovoltaicmodulematerialsandphotovoltaicarraysꎻAdoptingflexiblecombinationsofdifferenttypesofphotovoltaicsinconstructiontoderivemultipleapplicationscenariosꎻIntermsoffunctionalityꎬphotovoltaicpowergenerationismulti ̄dimensionallyintegratedwiththefunctionsoflightingꎬheatcollectionꎬshadingꎬandprotectionofbuildingenvelopesꎻIntermsofperformanceꎬthephotovoltaicorientationandtiltangleꎬheatdissipationdesignꎬandelectricalsystemareintegratedtoimproveproductionefficiency.Theanalysisprovidenewideasforthepromotionandapplicationofintegratedbuildingandphotovoltaictechnology.㊀㊀Keywords:BuildingIntegrationPhotovoltaic(BIPV)ꎻSolarDecathlonChina(SDC)ꎻzeroenergybuildingsꎻenergysavingandcarbonreduction8李涛ꎬ等:外显㊁灵活㊁多维㊁高效 从2022中国国际太阳能十项全能竞赛看建筑与光伏一体化设计趋势0 引言随着国家 双碳 目标的提出ꎬ建筑领域的节能减碳势在必行ꎮ建筑光伏一体化技术(BuildingIntegratedPhotovoltaicꎬBIPV)是实现建筑行业节能减碳的重要手段ꎮ随着各种光伏产品的推陈出新ꎬ建筑与光伏的一体化设计也出现了更多新的可能ꎮ国际太阳能十项全能竞赛(SolarDecathlonꎬSD)作为太阳能领域的奥林匹克 ꎬ对光伏与建筑一体化技术的示范和推广起到积极作用ꎬ历届参赛作品中的设计已成为BIPV的风向标ꎬ对我国BIPV的发展具有引领作用ꎮ建筑光伏一体化(BIPV)指的是将太阳能光伏发电产品集成或整合到建筑上ꎬ使它不但具有外围护结构的功能ꎬ同时又能产生电能供建筑使用ꎬ早期建筑与光伏结合的方式称为建筑附加光伏(BuildingAttachedPhotovoltaicꎬBAPV)[1]ꎮ与BAPV相比ꎬBIPV更容易整合到建筑的屋面㊁立面和各种构件中ꎬ高效地实现建筑与光伏的一体化设计ꎮ在当前倡导 绿色低碳的背景下ꎬBIPV已成为太阳能建筑设计和研究主流ꎮ王崇杰㊁李旻泽认为BIPV设计应该从光伏产品的特征出发[2ꎬ3]ꎮ石峰㊁邓丰指出BIPV设计应积极考虑单轴太阳能跟踪技术㊁PVT技术㊁光伏屋顶通风道以及PCM储热技术以提高系统工作效率[4ꎬ5]ꎮ张彦喆㊁张弘提出光伏组件尺寸与建筑空间尺寸进行模数化适配的BIPV设计方法[6ꎬ7]ꎮ杨向群㊁褚英男强调BIPV设计中能源技术与建筑艺术的结合[8ꎬ9]ꎮ师劭航认为BIPV立面设计应注重光伏产品㊁控制策略与整合设计[10]ꎮ总的来说ꎬ目前关于BIPV的研究将其作为太阳能建筑中的单项技术讨论ꎬ缺乏从建筑师视角对其进行多维度的系统研究ꎬ本文对中国国际太阳能十项全能竞赛中15个参赛作品中建筑与光伏一体化设计趋势进行系统的比较和分析(见表1)ꎬ为我国建筑与光伏一体化设计提供新思路ꎮ表1㊀SDC2022各赛队BIPV应用概况Table1BIPVapplicationoverviewofSDC2022建筑概况美学表现项目名称建筑外观BIPV应用部位朝向与倾角组件特征方阵形态构造整合功能复合性能提升散热方式系统类型Y-Projecta坡屋面南立面外遮阳棚S-22ʎS-90ʎS-0ʎ红棕色半透光钙钛矿一体融合式胶结密封采光顶透光幕墙室外遮阳自然对流+机械辅助通风自然对流普通并网离网一体系统24 35宅家b平屋面S-37ʎ黑色单晶硅组件阵列式框架架空-自然对流PV/T模块化可持续魔盒c平屋面露台顶S-45ʎ黑色碲化镉彩色半透光碲化镉组件阵列式重物压制框架架空造-露台遮阳自然对流普通并网离网一体系统极光之家d平屋面S-10ʎ黑色单晶硅整体架空预埋支座屋面遮阳自然对流普通并网离网一体系统草原方舟e坡屋面S-25ʎE-25ʎW-25ʎN-25ʎ不透光金属铝板色碲化镉一体融合式胶结密封屋面围护自然对流光伏发电+斯特林发电机HUIHOUSEf坡屋面S-37ʎ黑色铜铟镓硒光伏瓦叠合式光伏瓦金属屋面夹持块屋面排水自然对流+水冷PV/TPixelHouseg坡屋面阳光间雨棚院墙S-38ʎS-25ʎS-25ʎS-70ʎ均一黑色单晶硅黑色半透光碲化镉面层叠合式一体融合式--金属屋面夹持块胶结密封---采光+集热防护-自然对流PV/TT&A-Househ坡屋面走廊顶部走廊外墙S-45ʎS-0ʎS-45ʎ黑色单晶硅黑色半透光碲化镉黑色碲化镉面层叠合式整体架空百叶式框架架空胶结密封插挂式-遮阳自然对流普通并网离网一体系统R-CELLSi坡屋面S-14ʎS-20ʎ黑色单晶硅黑色碲化镉面层叠合式金属屋面夹持块-自然对流光伏发电+风力发电复合系统SMARTj坡屋面S-20ʎ黑色单晶硅面层叠合式金属钉固-自然对流普通并网离网一体系统斜屋k坡屋面S-37ʎ均一黑色单晶硅面层叠合式金属屋面夹持块屋面排水自然对流光储直柔系统9LITaoꎬetal.ExplicitꎬFlexibleꎬMultidimensionalandEfficient:TrendofBuildingandPhotovoltaicIntegratedDesignfromSolarDecathlonChina2022表1㊀SDC2022各赛队BIPV应用概况(续表)Table1BIPVapplicationoverviewofSDC2022(continuedtable)建筑概况美学表现项目名称建筑外观BIPV应用部位朝向与倾角组件特征方阵形态构造整合功能复合性能提升散热方式系统类型BBBCl平屋面庭院顶部S-5ʎ黑色单晶硅彩色半透光碲化镉组件阵列式整体架空金属屋面夹持块框架架空-庭院遮阳自然对流光储直柔系统SolarArkm异形屋面E/W-15ʎ黑色单晶硅组件阵列式框架架空屋面遮阳自然对流光伏发电+风力发电复合系统HopeLandn坡屋面东/西立面南采光顶南立面外窗E/W/S-15ʎE/S-90ʎN-15ʎS-90ʎE/W-90ʎ均一黑色单晶硅黑色半透光碲化镉面层叠合式一体融合式-胶结密封-屋面围护-采光自然对流普通并网离网一体系统栖居3 0o平屋面檐口露台栏板S-15ʎE/W/S-15ʎE/W/S-90ʎ黑色碲化镉黑色半透光碲化镉组件阵列式-框架架空--遮阳防护自然对流并网离网一体系统各项目外观照片1㊀美学表现外显建筑师有很长一段时间都认为光伏组件是影响建筑美学的负面因素ꎬ想方设法将其隐藏ꎬ但对于BIPV而言光伏组件已经脱离了简单的建筑发电设备范畴ꎬ越来越多的太阳能建筑开始积极显露光伏组件自身的特点ꎬ使其成为建筑美学的重要构成元素ꎮ1 1㊀组件色彩、纹理及形态目前ꎬ市场上主流的光伏电池有两类ꎬ一是晶体硅类的单晶硅电池和多晶硅电池ꎬ二是化合物薄膜类的碲化镉㊁铜铟镓硒及钙钛矿电池ꎮ从色彩纹理上看ꎬ由于电池材料和制备工艺的不同ꎬ晶硅光伏组件和化合物薄膜光伏组件的外观有着明显的差异ꎬ晶硅组件采用的是切片拼接工艺ꎬ光伏组件外观呈现补丁状ꎬ而薄膜电池采用的是内级联结构工艺ꎬ外观均一[11]ꎮ从形态上看ꎬ光伏组件一般为矩形平板ꎬ新研发的柔性光伏电池打破了组件形态的限制ꎬ使得BIPV的应用场景更加丰富ꎮ为了满足建筑的要求ꎬ光伏组件的外观也得到了升级ꎬ各种纹理化组件㊁透光组件㊁彩色组件以及曲面形态的光伏瓦在建筑中开始大规模应用(见图1)ꎮSDC2022竞赛中ꎬ各个作品结合自身的特点探索新型BIPV组件与建筑的结合ꎮ项目BBBC 是面向灾后紧急救援的建筑ꎬ其在内部庭院顶部应用了彩色透光的光伏组件ꎬ选用黄色㊁蓝色及透明色形成的图案肌理ꎬ不仅提升了建筑的第五立面ꎬ营造了丰富的光影效果ꎬ还能产生积极的心理暗示ꎬ安抚受灾人员的情绪(见表1㊁图l)ꎮ项目 HUIHOUSE 的原型是传统徽派建筑ꎬ 白墙黛瓦 是其风格的重要体现ꎬ因此该项目的南向坡屋面使用了铜铟镓硒光伏瓦作为光伏发电元件ꎬ将BIPV技术地融入了传统建筑元素之中(见表1图f)ꎮ1 2㊀BIPV方阵形态建筑上的光伏组件是以光伏方阵形态来呈现的ꎬ因此光伏方阵的形态设计对建筑的外观形象影响巨大ꎮ常见的BIPV设计中光伏方阵的形态主要分为以下几01李涛ꎬ等:外显㊁灵活㊁多维㊁高效 从2022中国国际太阳能十项全能竞赛看建筑与光伏一体化设计趋势图1㊀各种外观的光伏组件Fig.1Photovoltaicmoduleswithvariousappearances种:组件阵列式㊁整体架空式㊁面层叠合式㊁一体融合式ꎮ组件阵列式的BIPV形态中光伏组件呈多排锯齿韵律形态ꎬ组件较为接近屋面ꎬ施工布线方便ꎬ多用于平屋面ꎬ但需注意组件前后排的自遮挡ꎮ 阳光方舟3 0 屋顶的光伏方阵以东西朝向两块组件为一组形成阵列形态ꎬ成为双曲抛物面壳体建筑外观形象的主要构成部分ꎬ见图2(a)ꎻ整体架空式的BIPV形态中光伏组件在一个平面内纵横连续布置于屋面大型支撑系统ꎬ组件远离屋面ꎬ形成下部灰空间ꎬ施工布线方便ꎬ多用于平屋面ꎮ 极光之家 屋顶光伏为整体架空形态ꎬ平屋面上单晶硅组件方阵下部形成灰空间ꎬ可上人和放置设备ꎬ见图2(b)ꎻ面层叠合式的BIPV形态中光伏组件贴附在建筑围护结构表面ꎬ但不起围护作用ꎬ组件接近屋面ꎬ多用于坡屋面ꎬ但组件下部与屋面之间通风散热和布线空间较为狭小ꎮ 像素之家 屋顶为 三折式 的坡屋面ꎬ外侧叠合了单晶硅组件ꎬ光伏方阵与建筑形象的关系并不像 阳光方舟3 0 那样张扬ꎬ而是二者相互融合达到统一整体ꎬ见图2(c)ꎻ一体融合式的BIPV形态中光伏组件以玻璃幕墙或采光顶的形式应用到建筑上来ꎬ光伏组件成为建筑的实际围护结构ꎬ但需保证其构造的密封严实以及管线在室内外露的问题ꎮ 草原方舟建筑原型是游牧民族的蒙古包ꎬ将其毡顶转译为坡屋顶ꎬ融合碲化镉薄膜组件于坡屋顶实现产能与建筑形象的统一ꎬ见图2(d)ꎮ图2㊀SDC2022中的光伏方阵形态实例Fig.2ExampleofphotovoltaicarrayshapeinSDC2022㊀㊀可以看出ꎬ从建筑的美学表现上考虑BIPV技术可以从微观和宏观两个层面进行:在微观层面ꎬ从组件的色彩㊁纹理和形态入手确定光伏组件的选型ꎬ选择符合建筑外观和功能要求的光伏组件ꎬ展现建筑的文脉特征ꎻ在宏观层面ꎬ以建筑的空间特点出发确定光伏方阵形态ꎬ使其成为促进建筑形态美观性的积极要素ꎮ2㊀构造整合灵活随着技术的进步㊁光伏材料的丰富ꎬ 建筑 光伏 构造摆脱了BAPV式的大型支撑系统ꎬ不管是屋面光伏还是墙面光伏ꎬ都可以根据所应用部位的需求灵活地整合到建筑上来ꎮ2 1㊀光伏—屋面构造SDC2022中屋面光伏组件通过框架架空㊁预埋支座㊁金属屋面夹持块㊁重物压置㊁钉固㊁胶结密封等构造方式与建筑进行整合(见图3)ꎮ 24 35宅家 采用了框架架空构造ꎬ屋面光伏支撑系统的基础为一个架空于屋面的金属框架ꎬ不直接接触屋面ꎬ连接点牢固ꎬ但用钢量增加ꎬ比较适用平屋面ꎬ见图3(a)ꎻ 极光之家 采用预埋支座构造ꎬ将光伏组件支撑框架的基础部分预埋进屋面ꎬ然后再连接上部支撑结构ꎬ其11LITaoꎬetal.ExplicitꎬFlexibleꎬMultidimensionalandEfficient:TrendofBuildingandPhotovoltaicIntegratedDesignfromSolarDecathlonChina2022支座处防水薄弱ꎬ需特别加强ꎬ适用平屋顶ꎬ见图3(b)ꎻ R-CELLS 采用金属屋面夹持块构造ꎬ将金属屋面的立缝部位与相对应的金属夹持块结合用以固定和支撑光伏方阵ꎬ这种构造防水性能好ꎬ安装方便ꎬ但组件下部空间小ꎬ不利于散热及布线ꎬ见图3(c)ꎻ Y-Project 屋面采光顶采用胶结密封构造ꎬ在屋面表层布置一层金属龙骨ꎬ通过结构胶将光伏组件固定于金属龙骨上ꎬ最后使用密封材料将组件之间的缝隙填充平整ꎬ没有繁琐机械连接ꎬ但需要严格的密封措施ꎬ见图3(d)ꎻ SMART 采用钉固构造ꎬ将光伏檩条直接通过金属钉固定在屋面上ꎬ施工简单方便ꎬ但金属钉会穿过防水层ꎬ需要在檩条与屋面交接的部位进行密封处理ꎬ见图3(e)ꎻ 模块化可持续魔盒 采用物压置构造ꎬ光伏组件支撑构架上压置水泥块或者石块等重物ꎬ起到固定的作用ꎬ施工简单方便ꎬ但增加了屋面荷载ꎬ重物有坠落风险ꎬ适用于平屋面ꎬ见图3(f)ꎮ图3㊀SDC2022中的光伏-屋面构造实例Fig.3ExampleofPVroofstructureinSDC20222 2㊀光伏—墙面构造BIPV用的光伏组件为玻璃材质ꎬ因此建筑墙面的BIPV构造一般与玻璃幕墙类似ꎬ以金属横竖龙骨为框架ꎬ将框架与光伏组件通过结构胶粘接在一起ꎬ不需要金属卡具ꎮ本届SDC竞赛中ꎬ应用BIPV立面的应用类型比较全面ꎬ有明框幕墙㊁隐框幕墙㊁插挂式幕墙ꎬ幕墙嵌板材质既有透光幕墙也有非透光幕墙ꎮ Y-Project 的南立面架以明框幕墙的形式安装透光钙钛矿薄膜组件ꎬ见图4(a)ꎻ HopeLand 的南立面以隐框幕墙的形式安装透光碲化镉薄膜组件ꎬ见图4(b)ꎻ东㊁西立面则是隐框的不透光单晶硅组件幕墙ꎬ见图4(c)ꎻ T&A-House 的西立面则通过插挂式碲化镉薄膜组件构成ꎬ见图4(d)ꎮ㊀㊀不管是一体化的 光伏-屋面 构造还是 光伏-墙面 构造ꎬ其与建筑的构造整合方式极具灵活性ꎬ高效的整合方式使得各个构造的围护性能与组件发电效能相互促进ꎮ3㊀功能复合多维接受太阳辐射产生电能是光伏组件的基本功能ꎬ现阶段BIPV设计中的光伏组件已经突破了这单一功能ꎬ使其功能向各个维度拓展ꎬ如各种形式的光伏幕墙集合了围护㊁采光㊁发电等功能ꎬ各种形式的光伏集热空间㊁采光构造以及光伏建筑构件ꎮ21李涛ꎬ等:外显㊁灵活㊁多维㊁高效 从2022中国国际太阳能十项全能竞赛看建筑与光伏一体化设计趋势图4㊀SDC2022中的光伏-墙面构造实例Fig.4ExampleofPVwallstructureinSDC20223 1㊀BIPV幕墙光伏幕墙是BIPV功能复合最常见的形式ꎬ按嵌板类型分可以分为透光光伏幕墙与不透光光伏幕墙ꎮ透光光伏幕墙中采用透光光伏组件作为幕墙嵌板ꎬ有单㊁双层两种形式ꎮ单层透光光伏幕墙可以使一部分光线进入室内ꎬ提供室内采光ꎬ如项目 HopeLand 南立面以40%透光率的碲化镉薄膜组件作为幕墙嵌板ꎬ但组件发电时产生的热量也会进入室内ꎬ增加室内的热负担ꎬ见图5(a)ꎻ如果内侧加一层隔热玻璃ꎬ中间留通风散热空腔形成双层幕墙构造ꎬ可以有效地将发电余热排除到室外ꎬ如项目 Y-Project 南立面利用透光的钙钛矿薄膜组件作为外层幕墙嵌板ꎬ内侧为实体墙和窗户ꎬ见图5(b)ꎮ不透光光伏幕墙用不透光的光伏组件作为幕墙嵌板ꎬ一般为双层ꎬ如项目 HopeLand 东㊁西立面以不透光的单晶硅组件作为幕墙嵌板ꎬ上下留有通风孔洞ꎬ见图5(c)ꎮ图5㊀BIPV幕墙示意图及应用Fig.5SchematicdiagramandapplicationofBIPVcurtainwall3 2㊀BIPV集热空间Trombe墙和阳光房等被动式集热空间能够使房屋在冬季能够保持低能耗状态下的舒适度ꎬ将光伏组件应用于Trombe墙和阳光间形成的新型集热空间ꎬ不仅可以收集热量ꎬ还可发电ꎬ同时还可以将光伏组件发电时产生的有效废热利用起来ꎮPV-Trombe墙分为内置式和外置式两种类型[12ꎬ13]ꎬ内置式PV-Trombe墙的光伏组件位于内侧墙体表面ꎬ太阳光需要穿过外侧玻璃到达组件表面才能完成光电转换ꎬ这一过程会造成一定的效率衰减ꎻ外置式PV-Trombe墙的光伏组件位于外侧ꎬ要求光伏组件为透光组件以保证能有足够的太阳辐射进入墙体的蓄热体中ꎬ组件因透光也会造成发电效率的降低ꎮ光伏阳光间是通过将阳光间的玻璃替换成透光的光伏组件ꎬ如项目 PixelHouse 在南侧设置了集热阳光间ꎬ将其顶部为透光的碲化镉薄膜组件(见图6)ꎮ31LITaoꎬetal.ExplicitꎬFlexibleꎬMultidimensionalandEfficient:TrendofBuildingandPhotovoltaicIntegratedDesignfromSolarDecathlonChina2022图6㊀ PixelHouse 光伏阳光间Fig.6 PixelHouse PV-sunshineroom3 3㊀BIPV采光构造利用透光组件作为窗扇ꎬ可以进行采光ꎬ但对视线会造成一定遮挡ꎬ比较适用于高窗㊁天窗等对视线要求不高的区域ꎮ项目 HopeLand 在东西立面都将40%透光率的碲化镉薄膜组件用于固定的窗扇ꎬ具有良好的采光效果ꎬ见图7(a)ꎻ屋顶区域也通过透光光伏组件实现了采光天窗的应用ꎬ见图7(b)ꎮ图7㊀BIPV采光构造示意图及应用Fig.7SchematicdiagramandapplicationofBIPVdaylightingstructure3 4㊀其他功能光伏组件还可以应用在建筑的防护构件与遮阳构件上(见图8)ꎮ防护构件常见的应用场景有光伏图8㊀光伏遮阳及防护构件示意图Fig.8Schematicdiagramofphotovoltaicshadingandprotectivecomponents雨蓬和光伏栏板ꎬ遮阳构件应用较为多样ꎬ主要有外窗固定光伏遮阳板㊁光伏百叶以及光伏遮阳棚等ꎮ4㊀性能提升高效如何提高光伏组件的发电效率是光伏行业面临的一个难题ꎮ结合不同的应用场景ꎬBIPV系统通过诸多手段提高自身的效率:首先是基于资源的光伏倾角㊁方位角设计ꎬ其次是基于方阵形式和构造的散热设计ꎬ第三是综合的能源系统设计ꎮ4 1㊀倾角朝向设计在光伏方阵的设计中ꎬ往往会以当地的最佳倾角和最佳朝向安装光伏组件ꎬ使其倾斜面上的年总辐射量达到最大ꎬ SDC2022 竞赛的15个赛队的建筑为了迎合其形态的要求ꎬ光伏组件的安装倾角与方位角的差别比较大ꎮ为了探索项目所在地 张家口市张北县光伏组件布置的最佳倾角与方位角ꎬ选取东㊁西㊁南㊁北㊁东南㊁东北㊁西南㊁西北八个朝向ꎬ分别以0ʎ~90ʎ每5ʎ为一个计算点利用Grasshopper和Lady ̄bug计算全年太阳辐射ꎬ计算结果见图9ꎮ从朝向来看ꎬ正南为最佳ꎬ西南次之ꎻ从倾角来看ꎬ正南㊁西南朝向的最佳倾角均为35ʎ左右ꎮ总体来说ꎬ在张家口地区光伏组件的布置应以贴近正南朝向为佳ꎬ组件倾角应在35ʎ左右ꎮ4 2㊀散热设计光伏电池的输出性能会随着温度的升高而降低ꎬ因此必须做散热设计保证其效率ꎮ光伏组件产生的热量一般都通过自然对流的方式排散到室外环境中ꎬ当自然对流无法满足组件的散热需求时ꎬ可以通过机械设备主动散热(见图10(a)~(e))ꎮ自然对流散热41李涛ꎬ等:外显㊁灵活㊁多维㊁高效 从2022中国国际太阳能十项全能竞赛看建筑与光伏一体化设计趋势图9㊀张家口光伏各朝向及其最佳倾角的太阳辐射Fig.9SolarradiationatvariousorientationsandinclinationsofPVinZhangjiakou是通过自然通风将光伏组件背部的热量带走ꎬ这种方式最为简单㊁经济ꎮ相对于面层叠合式的BIPV形态ꎬ组件阵列式和整体架空式都具有很高的开放程度ꎬ组件背部直接暴露在室外空气中ꎬ完全可以利用自然通风达到散热的目的ꎮ面层叠合式的BIPV形态中会在屋面与光伏组件之间形成一个空腔ꎬ组件散热效率一定程度上取决于空腔的高度[14ꎬ15]ꎬ但过高的通风层高度会对BIPV的整体形态造成影响ꎮ机械主动散热方式比较适用于面层叠合式的BIPV形态ꎬ通过风机加强空腔内部的对流以达到散热的目的ꎬ也可以通过低温介质在空腔内部循环带走热量ꎬ这两种方式在运行的时候都会消耗一定量的电能ꎬ但使组件发电效率提高后多出的发电量会反哺这部分消耗ꎬ再者还可以通过一些技术手段将这部分余热利用起来ꎮ合肥工业大学的 HUIHOUSE 在光伏瓦的下部空腔里布置导热铜管ꎬ铜管内部通水流ꎬ通过水流将光伏瓦产生的热量收集起来ꎬ供建筑的日常使用ꎬ既能帮助光伏组件散热ꎬ又能实现太阳能光伏系统 热电联产 的高效利用ꎬ见图10(f)ꎮ图10㊀BIPV散热示意图Fig.10BIPVheatdissipationdiagram4 3㊀系统设计在SDC2022竞赛中ꎬ各赛队积极探索使用新型高效的电气系统ꎮ为了削减夏季空调负荷峰值㊁缓解电网增容压力㊁增强电网供电可靠性ꎬ BBBC 项目应用了光储直柔低压直流供配电系统:在光伏布置方面ꎬ屋顶布置了51块双面双玻单晶硅组件ꎬ全年产能可达到31920~35400kW hꎬ每年可反哺电网17040~20520kW hꎻ在储能方面ꎬ配置了6块电池ꎬ可存储28 8kW h的电量ꎻ在电气系统的配置上ꎬ该项目拥有交流配电与直流配电两套系统ꎬ其中交流配电以220V市政电为主ꎬ供给建筑内部的交流电器ꎬ直流配电有375V和超低压48V两种供电模式ꎬ其中375V直接用于空调和地暖等大功率设备的供电ꎬ48V低压直流电用于电视㊁LED灯具㊁电脑等低压设备ꎻ在柔性用能方面ꎬ可以通过软件实现对储能电池的充放电控制㊁逆变器的启闭状态以及直流负荷的功率ꎬ降低了电流转换的损耗ꎬ提高了用电安全ꎬ见图11(a)ꎮ R-CELLS 项目根据张家口地区的丰富的太阳能与风能资源ꎬ可再生能源系统应用了风光互补的复合发电系统:该系统中光伏发电部分以屋顶的90片单晶硅组件和98块碲化镉薄膜组件为主ꎬ总装机容量为35 14kWpꎬ年发电量可达49776kW hꎻ风力发电部分主要靠建筑延伸屋檐开孔处的5台螺旋风机ꎬ风机单台功率为400Wꎬ输出电压为直流240Vꎬ装机容量为2 0kWꎬ不仅可以利用风力发电ꎬ还减轻了风荷载对屋面的作用ꎬ见图11(b)ꎮ光伏光51LITaoꎬetal.ExplicitꎬFlexibleꎬMultidimensionalandEfficient:TrendofBuildingandPhotovoltaicIntegratedDesignfromSolarDecathlonChina2022热一体化(PV/T)系统不仅可以发电ꎬ还可以获得相应的热收益ꎬ同时降低了光伏组件的温度ꎬ提高系统的综合能量利用效率[16]ꎮ项目 PixelHouse 在屋顶安装了12 09kW的PV/T组件ꎬ年产热量约为31329kW hꎬ其内部采用相变材料作为换热工质ꎬ通过相变材料将光伏组件产生的废热收集与地下的储热水箱ꎬ水箱中为低温热水ꎬ低温热水经电加热后提高温度用于盥洗㊁淋浴以及地暖供热ꎬ见图11(c)ꎮ图11㊀SDC2022新型光伏系统Fig.11NewphotovoltaicsysteminSDC20225 结语建筑光伏一体化技术是实现 零能耗建筑 的重要途径ꎬ越来越多的建筑将通过这一技术从 耗能 迈向 产能 ꎬ但该技术并不是将建筑和太阳能光伏技术二者的简单叠加ꎬ还需要考虑二者的融合性与高效性ꎮ本文通过对SDC2022竞赛案例的分析ꎬ总结了BIPV的设计趋势与要点:(1)BIPV在美学表现上积极外显ꎬ在建筑外观上强调光伏组件自身和光伏方阵形态的美学特点ꎬ因此BIPV设计要通过光伏组件的颜色㊁纹理及方阵的形态展示建筑特色ꎬ使光伏的应用与建筑的风格协调一致ꎻ(2)BIPV在构造整合方面十分灵活ꎬ各种各样的构造方式随着繁多的组件产品和不同的建筑应用部位应运而生ꎬ因此在光伏组件与建筑进行一体化集成时灵活选择适宜的构造方式ꎻ(3)BIPV设计中光伏组件的功能维度开始拓展ꎬ突破单一发电设备的功能ꎬ成为融合围护㊁采光㊁集热㊁遮阳㊁防护等的多功能建材ꎬ因此BIPV设计要结合建筑要求ꎬ充分发挥光伏组件复合功能ꎬ提升应用潜力ꎻ(4)BIPV系统性能提升方法越来越高效ꎬ除了通过优化光伏方阵的朝向与倾角以外ꎬ还可从散热设计和电气系统来提高整个光伏系统的产能效率ꎬ因此BIPV设计需要结合当地的气候与环境与资源状况ꎬ积极结合其他可再生能源ꎬ实现多能互补ꎬ优化系统ꎬ最大程度发挥光伏组件的发电潜力ꎮ致谢:㊀㊀感谢 BBBC ㊁ R-CELLS ㊁ PixelHouse 团队提供图11资料ꎮ参考文献:[1]龙文志.太阳能光伏建筑一体化[J].建筑节能ꎬ2009ꎬ37(7):1-9.[2]王崇杰ꎬ张泓ꎬ房涛ꎬ等.太阳能住宅建筑设计策略研究 以2018中国国际太阳能十项全能竞赛为例[J].建筑节能ꎬ2019ꎬ47(7):60-65.[3]李旻泽.基于光伏特性的光伏建筑一体化景观设计策略研究 以中国(青海)太阳能光伏展示馆建设项目为例[J].智能建筑与智慧城市ꎬ2022ꎬ(9):121-123.[4]石峰ꎬ乐乐.相变材料在零能耗太阳能住宅中的应用研究 以国际太阳能十项全能竞赛作品为例[J].建筑节能ꎬ2019ꎬ47(3):35-40.[5]邓丰ꎬ谭洪卫.零能耗住宅设计的技术路线研究 以同济大学三届国际太阳能十项全能竞赛(SD)作品为例[J].城市建筑ꎬ2015ꎬ(31):44-47.[6]张彦喆ꎬ张健.新农村城镇化绿色宜居建筑探索 2013年国际太阳能十项全能竞赛 上海交通大学作品[J].华中建筑ꎬ2014ꎬ32(7):70-76.[7]张弘ꎬ李珺杰ꎬ董磊.零能耗建筑的整合设计与实践 以清华大学O-House太阳能实验住宅为例[J].世界建筑ꎬ2014ꎬ(1):114-117.[8]杨向群ꎬ高辉.零能耗太阳能住宅建筑设计理念与技术策略 以太阳能十项全能竞赛为例[J].建筑学报ꎬ2011ꎬ(8):97-102.[9]褚英男ꎬ宋晔皓ꎬ孙菁芬ꎬ等.近零能耗导向的光伏建筑一体化设计路径初探[J].建筑学报ꎬ2019ꎬ(S2):35-39.[10]师劭航ꎬ褚英男ꎬ高唯芷ꎬ等.建筑光伏一体化立面技术应用思考[J].世界建筑导报ꎬ2022ꎬ37(4):35-38.[11]汤洋ꎬ秦文军ꎬ王璐.欧洲光伏建筑一体化发展研究[J].建筑学报ꎬ2019ꎬ(S2):10-14.[12]马杨ꎬ季杰ꎬ孙炜ꎬ等.2种PV-Trombe墙的光电光热性能对比研究[J].太阳能学报ꎬ2019ꎬ40(8):2323-2329.[13]季杰ꎬ易桦ꎬ何伟ꎬ等.PV新型Trombe墙光电光热性能数值模拟[J].太阳能学报ꎬ2006ꎬ(9):870-877.[14]张瑞ꎬ郝国强ꎬ于霄童ꎬ等.光伏建筑屋顶的通风腔设计分析[J].建筑科学ꎬ2013ꎬ29(4):65-72.[15]范昕杰.基于坡屋顶光伏板通风腔的散热仿真实测分析[J].建筑热能通风空调ꎬ2016ꎬ35(10):21-25.[16]陈金峰ꎬ代彦军.建筑构件化太阳能光伏/光热(PV/T)器件及其在建筑中的应用[J].建设科技ꎬ201ꎬ5(8):53-55.ә作者简介(通讯作者):李涛(1984)ꎬ男ꎬ陕西咸阳人ꎬ毕业于天津大学ꎬ建筑学专业ꎬ博士ꎬ副教授ꎬ主要从事绿色建筑设计方面的研究(182171912@qq.com)ꎮ61。
综合能源系统关键技术综述与展望
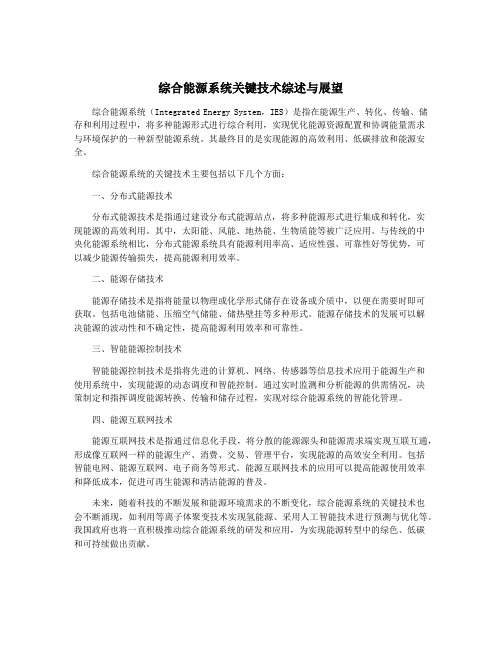
综合能源系统关键技术综述与展望综合能源系统(Integrated Energy System,IES)是指在能源生产、转化、传输、储存和利用过程中,将多种能源形式进行综合利用,实现优化能源资源配置和协调能量需求与环境保护的一种新型能源系统。
其最终目的是实现能源的高效利用、低碳排放和能源安全。
综合能源系统的关键技术主要包括以下几个方面:一、分布式能源技术分布式能源技术是指通过建设分布式能源站点,将多种能源形式进行集成和转化,实现能源的高效利用。
其中,太阳能、风能、地热能、生物质能等被广泛应用。
与传统的中央化能源系统相比,分布式能源系统具有能源利用率高、适应性强、可靠性好等优势,可以减少能源传输损失,提高能源利用效率。
二、能源存储技术能源存储技术是指将能量以物理或化学形式储存在设备或介质中,以便在需要时即可获取。
包括电池储能、压缩空气储能、储热壁挂等多种形式。
能源存储技术的发展可以解决能源的波动性和不确定性,提高能源利用效率和可靠性。
三、智能能源控制技术智能能源控制技术是指将先进的计算机、网络、传感器等信息技术应用于能源生产和使用系统中,实现能源的动态调度和智能控制。
通过实时监测和分析能源的供需情况,决策制定和指挥调度能源转换、传输和储存过程,实现对综合能源系统的智能化管理。
四、能源互联网技术能源互联网技术是指通过信息化手段,将分散的能源源头和能源需求端实现互联互通,形成像互联网一样的能源生产、消费、交易、管理平台,实现能源的高效安全利用。
包括智能电网、能源互联网、电子商务等形式。
能源互联网技术的应用可以提高能源使用效率和降低成本,促进可再生能源和清洁能源的普及。
未来,随着科技的不断发展和能源环境需求的不断变化,综合能源系统的关键技术也会不断涌现,如利用等离子体聚变技术实现氢能源、采用人工智能技术进行预测与优化等。
我国政府也将一直积极推动综合能源系统的研发和应用,为实现能源转型中的绿色、低碳和可持续做出贡献。
计及综合需求响应的综合能源系统优化调度
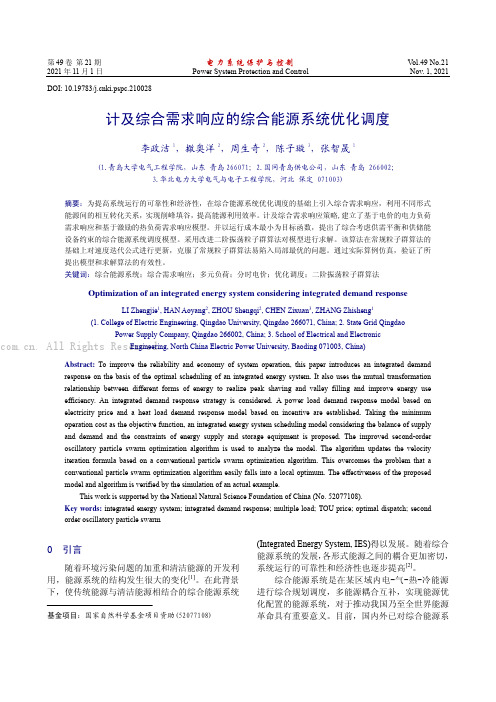
第49卷第21期电力系统保护与控制Vol.49 No.21 2021年11月1日Power System Protection and Control Nov. 1, 2021 DOI: 10.19783/ki.pspc.210028计及综合需求响应的综合能源系统优化调度李政洁1,撖奥洋2,周生奇2,陈子璇3,张智晟1(1.青岛大学电气工程学院,山东 青岛266071;2.国网青岛供电公司,山东 青岛 266002;3.华北电力大学电气与电子工程学院,河北 保定 071003)摘要:为提高系统运行的可靠性和经济性,在综合能源系统优化调度的基础上引入综合需求响应,利用不同形式能源间的相互转化关系,实现削峰填谷,提高能源利用效率。
计及综合需求响应策略,建立了基于电价的电力负荷需求响应和基于激励的热负荷需求响应模型。
并以运行成本最小为目标函数,提出了综合考虑供需平衡和供储能设备约束的综合能源系统调度模型。
采用改进二阶振荡粒子群算法对模型进行求解。
该算法在常规粒子群算法的基础上对速度迭代公式进行更新,克服了常规粒子群算法易陷入局部最优的问题。
通过实际算例仿真,验证了所提出模型和求解算法的有效性。
关键词:综合能源系统;综合需求响应;多元负荷;分时电价;优化调度;二阶振荡粒子群算法Optimization of an integrated energy system considering integrated demand responseLI Zhengjie1, HAN Aoyang2, ZHOU Shengqi2, CHEN Zixuan3, ZHANG Zhisheng1(1. College of Electric Engineering, Qingdao University, Qingdao 266071, China; 2. State Grid QingdaoPower Supply Company, Qingdao 266002, China; 3. School of Electrical and ElectronicEngineering, North China Electric Power University, Baoding 071003, China)Abstract: To improve the reliability and economy of system operation, this paper introduces an integrated demand response on the basis of the optimal scheduling of an integrated energy system. It also uses the mutual transformation relationship between different forms of energy to realize peak shaving and valley filling and improve energy use efficiency. An integrated demand response strategy is considered. A power load demand response model based on electricity price and a heat load demand response model based on incentive are established. Taking the minimum operation cost as the objective function, an integrated energy system scheduling model considering the balance of supply and demand and the constraints of energy supply and storage equipment is proposed. The improved second-order oscillatory particle swarm optimization algorithm is used to analyze the model. The algorithm updates the velocity iteration formula based on a conventional particle swarm optimization algorithm. This overcomes the problem that a conventional particle swarm optimization algorithm easily falls into a local optimum. The effectiveness of the proposed model and algorithm is verified by the simulation of an actual example.This work is supported by the National Natural Science Foundation of China (No. 52077108).Key words: integrated energy system; integrated demand response; multiple load; TOU price; optimal dispatch; second order oscillatory particle swarm0 引言随着环境污染问题的加重和清洁能源的开发利用,能源系统的结构发生很大的变化[1]。
CCHP_冷热电三联供技术
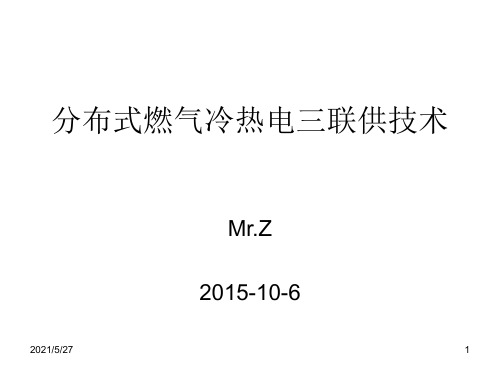
高能源利用效率、改善能源产业的安全性、解决环境污染,单一的大电网集中供电 解决 上述问题存在困难,而分布式供电系统恰好可以在提高能源利用率、改善安
全性与解决环境污染方面做出突出的贡献。因此,大电网与分散的小型分布式供电 方式的合理结合,被全球能源、电力专家认为是投资省、能耗低、可靠性高的灵活 能源系统,成为二十一世纪电力工业的发展方向。
目前市电平均价格,单独发电是不经济的。对于热负荷变化较大的建筑物或者负
荷率很低的场所,能源综合利用效率一般很难达到期望的效果,并且发电机的使
用寿命也会受到影响。
2.系统成本的经济性受政府行为干预的影响大。
CCHP成本中燃料占67%~78%,其经济效益受市场燃料与用电价格(电价、
气价、热价)的影响(希望的大趋势是电价上涨、气价下跌),这些与政府定价
分布式燃气冷热电三联供技术
Mr.Z
2015-10-6
2021/5/27
1
0 前言
分布式燃气冷热电联供系统(DES/CCHP)是一种建立在能量梯 级利用概念基础上,以天然气为一次能源,同时产生电能和可用热 (冷)能的分布式供能系统。
作为能源集成系统(Integrated Energy Systems),冷热电联供 系统按照功能可分成三个子系统:动力系统(发电)、供热系统(供 暖、热水、通风等)和制冷系统(制冷、除湿等)。目前多采用燃气 轮机或燃气内燃机作为原动机,利用高品位的热能发电,低品位的热 能供热和制冷,从而大幅度提高系统的总能效率,降低了燃气供应冷 热电的成本。联供技术的具体应用取决于许多因素,包括:电负荷大 小,负荷的变化情况、空间的要求、冷热需求的种类及数量、对排放 的要求、采用的燃料、经济性和并网情况等。
区域综合能源系统两阶段鲁棒博弈优化调度

DOI: 10.3785/j.issn.1008-973X.2021.01.021区域综合能源系统两阶段鲁棒博弈优化调度李笑竹,王维庆(新疆大学 可再生能源发电与并网技术教育部工程研究中心,新疆 乌鲁木齐 830047)摘 要:在区域综合能源系统的基本架构上,为了提升系统经济性与可再生能源并网能力,研究混合储能、冷热电联供机组(CCHP )、能量转换装置在多能互补下的两阶段优化运行模型. 利用虚拟能量厂(VEP ),平抑发、用电不确定性;采用鲁棒理论,构建灵活调整边界的不确定合集;引入条件风险理论,构建考虑多种不确定关系耦合下基于Copula-RCVaR 的能量管理风险模型. 针对上述模型特点,提出基于滤子技术的多目标鲸鱼算法进行求解. 分析不同可再生能源渗透率及集群效应对系统收益结果和运行策略的影响. 结果表明,引入虚拟能量厂可以提高利润1.9%,在保证稳定运行的前提下合理选择荷、源不确定变量的置信概率,可以提高利润5.9%.关键词: 区域综合能源系统(RIES );鲁棒理论;两阶段优化;收益损失风险;多目标优化算法中图分类号: TM 73 文献标志码: A 文章编号: 1008−973X (2021)01−0177−12Bi-level robust game optimal scheduling of regionalcomprehensive energy systemLI Xiao-zhu, WANG Wei-qing(Engineering Research Center of Ministry of Education for Renewable Energy Generation and Grid Connection Technology ,Xinjiang University , Urumqi 830047, China )Abstract: The two-stage optimal operation strategy model of hybrid energy storage, combined cooling, heating andpower (CCHP) units and energy conversion device was analyzed based on the basic framework of regional integrated energy system (RIES) in order to improve the system economy and the grid connection capacity of large-scale connected renewable energy under the RIES with multiple energy complementary. Virtual energy plant (VEP) was used to stabilize the uncertainty of power generation and consumption. The robust theory was used to construct the uncertain aggregate to adjust the boundary flexibly, and conditional risk theory was introduced to construct the risk model of RIES energy management based on Copula-RCVaR. A multi-objective whale optimal algorithm based on filter technology was proposed to solve the above complex model. The influence of different renewable energy penetration rate and their cluster effect on the income result and operation strategy of RIES was analyzed. Results show that the profit of RIES can be increased by 1.9% by introducing VEP. The profit of RIES can be increased by 5.9% by selecting a reasonable confidence probability of the uncertain variables for load and source based on the premise of ensuring the stable operation.Key words: regional integrated energy system (RIES); robust theory; bi-level optimization; revenue and loss risk; multi-objective optimization algorithm天然气的冷热电联供系统(combined cooling,heating and power ,CCHP )是连接电网与气网的耦合系统,也是区域综合能源系统(regional integ-rated energy system ,RIES )中最具发展前景的一种运营模式[1]. 目前,RIES 的研究多以优化不同效益目标,得到系统各设备的运行策略为主. Wei 等[2]收稿日期:2020−05−19. 网址:/eng/article/2021/1008-973X/202101021.shtml基金项目:国家自然科学基金资助项目(51667020,52067020);新疆自治区实验室开放课题资助项目(2018D4005).作者简介:李笑竹(1990—),女,博士生,从事电力系统能量管理及经济调度等研究. /0000-0003-0443-0449.E-mail :****************通信联系人:王维庆,男,教授. /0000-0001-6520-5507. E-mail :************.cn第 55 卷第 1 期 2021 年 1 月浙 江 大 学 学 报(工学版)Journal of Zhejiang University (Engineering Science)Vol.55 No.1Jan. 2021构建电转气的峰值负荷转移模型,从理论上证明了电气耦合系统具有较好的削峰填谷的效果;Guandalini等[3]对电气耦合系统进行了评价,结果证明,该系统可以提高可再生能源的可调度性;张儒峰等[4]提出合理利用弃风的电-气综合能源系统,实现互联系统之间的双向耦合;Qu等[5]利用电转气实现电力系统与天然气系统的双向能量流动,是促进风电消纳平滑功率需求的有效途径.上述文献均未考虑发、用电波动性对系统带来的收益损失风险. 张虹等[6-8]引入CVaR计算一定收益下系统要承担的收益风险,但均将CVaR转化为离散情况下最差CVaR进行求解,该方法结果的主观性强. 上述研究均仅考虑单维不确定变量,不适用于同时考虑多种不确定关系耦合下的建模与分析.综合需求响应利用冷热负荷的惯性特征,是平衡新电改下各市场主体利益诉求的绝佳手段[9],但RIES中考虑需求响应的调度方法较少涉及. 张虹等[6]让需求侧互动资源主动提供用电意愿,根据系统调度灵活选择用电行为;王文超等[9]将电价型需求响应应用于系统优化运行中;徐业琰等[10]通过电价型、激励型和博弈方法协同作用,实现对用户侧的联合调度. 来自于荷、源双侧(如风电、光伏、负荷等)的多重不确定性是RIES运行时面临的主要挑战. 在描述发电与用电不确定性上,场景法[11]、点估计法[12]、随机机会约束规划[13]、模型预测[14]都有较好的应用,但随机法与点估计法均需要实际中的大量样本数据,且场景法结果受场景个数的制约,机会约束规划难以保证求解效率与精度.鉴于以上分析,本文建立基于Copula-RCVaR 的区域综合能源系统两阶段鲁棒博弈优化调度模型. Copula-RCVaR模型能够对多个不确定变量耦合、不同决策需求下系统的收益损失风险进行分析与评估. 考虑综合需求响应,利用CCHP机组和虚拟能量厂(virtual energy plant,VEP)平抑RIES 内发电、用电波动性. 采用鲁棒理论,对系统内不确定变量建立不确定性合集,剖析不确定变量与系统经济性、保守性的动态相依关系,探索在不同决策需求下最经济可靠的调度方案. 针对模型特点,利用基于滤子技术的多目标鲸鱼算法进行求解. 以修改的IEEE33节点配电网与CCHP系统耦合形成RIES为例,验证模型能够在保证安全稳定的前提下,平衡各层主体利益,实现电力经济的可持续性发展.1 RIES的建模1.1 RIES的结构及运行方式如图1所示为RIES结构及运行方式示意图.在经典CCHP系统组成的RIES中,加入能量集线器与冷/热/电储能装置. 燃汽轮机是系统中的主要源动设备,发电量与RIES在能量交易中心向上级电网的购电量(包括在日前市场与实时市场的购电)共同承担系统负荷用电,通过余热转换装置与锅炉向系统内用户提供热负荷需求,系统的冷负荷需求由电制冷机和吸收式制冷机提供,电制冷机由电能驱动,吸收式制冷机由热能驱动.系统中,内燃气轮机和锅炉运行所需的天然气由RIES在能量交易中心向上级气网购得(仅在实时市场). 为了减少天然气的消耗,在系统中加入可再生能源电站(图1中的风电场),承担系统内部分电负荷与热负荷需求,可再生能源发电不接受调控且不计发电成本. RIES在日前市场向上级电网购买电量,能量盈余或亏空通过实时市场与上级电、气网的能量交换,调控CCHP机组组风电场图 1 RIES结构及运行示意图Fig.1 Structure and operation of RIES178浙江大学学报(工学版)第 55 卷合出力、VEP 、各能量转换装置得到平衡. 如图1所示,VEP 包括各储能系统与各种类型的可控负荷. 其中可控负荷根据特性分为以下4类[15]. 1)常规负荷(CL ),具有较大的随机性与波动性,且不可调控. 2)迎峰负荷(LSI ),切负荷量较低,一般为该类型总量的15%,补偿价格指数较高. 3)避峰负荷(LSII ),该类型负荷用电灵活性较大,切负荷量较高,为总量的30%,且补偿价格指数较低. 以上3种类型仅有电负荷CL-e/LSI-e/LSII-e. 4)可转移负荷(TL ),在不影响使用舒适度的前提下转移,补偿价格系数较低,但转移前、后的负荷总量不变,分为TL-h/TL-c ,表示热/冷负荷.该模型将RIES 与VEP 作为电力系统中不同的市场主体,针对运营体系及特点,采用双层多目标鲁棒优化对混合系统进行建模. 其中RIES 位于上层,VEP 位于下层. 优化时,先由RIES 向VEP 发送调度计划,VEP 在满足自身运行约束的前提下调控管辖内的可控资源(各储能系统、可控负荷)对该计划实行初步响应;将自身优化的结果反馈至上层,RIES 根据反馈结果进一步调整计划. 过程中,上、下两层信息互相更新与传递,在尽可能满足各系统电力需求的前提下,经济性、社会性最好. CCHP 机组与VEP 的参与可平抑发电与用电的波动性,将盈余电量在实时市场较稳定地外送,使RIES 获利,该运营模式在一定程度上可以提高可再生能源的并网能力. 由于冷/热网中的冷/热惯性,使得冷/热负荷中的不确定性能够被各自传输管道中的管存能力缓解[16],模型只考虑发、用电不确定性.1.2 CCHP 建模CCHP 装置互相耦合,与上级电、气网共同实现对RIES 能源的供应,各装置按如下方式建模.1)燃汽轮机. 出力与耗气量为二次函数.y GT t P e GT t G GT t y GT t 式中:a 1、b 1、c 1为燃汽轮机的耗气常数;、、为t 时刻燃气轮机的运行状态变量、出力和耗气量,其中=1为运行. 燃气轮机应满足P e GT min P e GT max R e GT U R e GT D T on GT T o ffGT式(2)为发电功率约束,式(3)为爬坡约束,式(4)为最小启停时间约束. 式中:、为出力上、下界限;、分别为向上和向下最大爬坡功率,、分别为最小开机和停机时间.2) 余热回收装置. 该装置输出热量与燃汽轮机的出力有关:P h WHR t 式中:a 2、b 2、c 2为耗量系数,为转换的可用热量.3) 电制冷机、吸收式制冷机和锅炉.P c ASR t P e ASR t P c ABS t P h ABS t P h B t G B t 式中:ηABS 、ηASR 分别为吸收式制冷机与电制冷机的效率,ηB 为锅炉热效率,、分别为电制冷机的制冷量与耗电量,、分别为吸收式制冷机的制冷量与吸热量,、分别为锅炉的1.3 数学模型1.3.1 上层模型 C e b C re tP e L t P h L t P c L t P e −s L t P VEP −e i ,tP VEP −h i ,t P VEP −c i ,t式中:ηt 为日前市场电量购买比例;C t S-e 、C t S-h 、C t S-c分别为电、热、冷能出售价格;、分别为日前、实时市场向上级电网的购电价格;C g 为向上级气网的购气价格;、、分别为t 时刻电、热、冷负荷;为电负荷的预测值,、、为RIES 对第i 个VEP 下达的调度计划,上第 1 期李笑竹, 等:区域综合能源系统两阶段鲁棒博弈优化调度[J]. 浙江大学学报:工学版,2021, 55(1): 177–188.179P VEP −e i ,tP grid t标e 、h 、c 表示VEP 类型,>0表示向系统注入能量;为系统与上级电网之间的电量交换,P t grid >0表示RIES 向上级电网售电,反之为购电;C VEP 为VEP 运行成本,由下层模型计算得出返回至上层;C conv 、C ek 为转换装置运行成本及旋转备N e LK W i K L i γW i γL i P u W i ,t P e −u L i ,t 式中:S WHR 、S ABS 、S ASR 分别为余热回收装置、吸收式制冷机、电制冷机的成本系数,N W 、分别为风电站及常规电负荷总数,/、/、Δ/Δ分别为风电/常规电负荷的旋转备用惩罚系数、功率偏差系数及各功率偏差上限.2)目标函数2. RIES 的收益损失风险最小值min f 1.2,详细见2章的风险能量管理模型,此处不赘述.P VEP −e i ,tP VEP −h i ,tP VEP −c i ,tN e v N h v N c v式中:、、分别为通过下层模型优化返回至上层,第i 个虚拟电、热、冷厂在t时刻的调度功率;、、分别为各虚拟电、热、冷厂的个数.上层模型除式(2)~(4)、(7)外,还需满足如P grid tP grid max P gridmin 为了防止RIES 与上级电网之间的联络线功率毛刺过多,使其能够运行平稳,将离散成10的整数倍,设置上、下功率界限为、,最大爬升功率为120 kW ,最小保持功率时间为2 h.1.3.2 下层模型 VEP 将RIES 下达的调用计划分解至各个可控单元上,使得两层之间的调度计划偏差最小,VEP 达到最大的经济效益与社会效益.1)目标函数1. 调度计划偏差最小.P VEP −e i ,t P VEP −h i ,t P VEP −c i ,t式中:、、由上层模型优化所得并传递至下层.2)目标函数2. 经济效益最好,调度成本最小.3)目标函数3. 社会效益最高,受文献[15]中以用电舒适度表征虚拟电厂社会效益方式的启发,以用能舒适度来表征VEP 的社会效益,即负荷切出率和转移率较低,社会效益较好.λe LSI λe LSII λh LT λc LT Lim LSI i ,max Lim LSII i ,max P max −h LT i P max −c LT iP e LSI i ,t P e LSII i ,t P h LT i ,t P c LT i ,t 式中:、、、分别为各类型负荷占该类总负荷比,、、、分别为各类型可控负荷的总量. 从式(17)可以看出,min f 2,3的取值为[0, 1.0],当各可控负荷在调度周期内完全不调用时,、、、均180浙 江 大 学 学 报(工学版)第 55 卷为0,此时用电舒适度最高,min f 2,3=1;当各可控负荷调度总量达到上限时,用电舒适度最低,min f 2,3=0.下层优化模型须满足各储能系统的相关约束. 其中储电约束如下.P e −ch ESS tP e −diss ESS t ρe ESS ηe c ηe d式中:SOC min 、SOC max 分别表示最小、最大充电状态,、分别为最大充、放电功率,、、分别为自放电率、充电率、放电率.储冷储热系统运行方式相同,储热为例,约束如下:P h −ch ESS t P h −diss ESS t 式中:、为最大充放电量.LSI 、LSII 运行方式类似,以LSI 为例,运行约束如下:可转移的冷热负荷运行类似,以热负荷为例:2 风险能量管理建模对1.3.1节上层模型的目标函数2进行建模. 鉴于发电、用电的不确定性,RIES 收益具有风险特征.2.1 CVaR 理论概述CVaR 度量损失的平均情况可以描述尾部风险[6],CVaR 为式中:E (.)为期望函数;x ∈ΩD 为决策变量;y ∈ΩR 为随机变量,概率密度函数为f PDF (y ),f c-l (x ,y )为RIES 的收益损失函数,且E (|f c -l (x ,y )|)<+∞;C α为损失值的阈值;VaR 为在给定置信度β下,RIES 可能遭受的最大损失值. 引入辅助函数计算CVaR ,表示如下:式中:[t ]+=max {t , 0}.2.2 Copula 函数在RIES 中,考虑发电与用电的双重不确定性,根据Copula 函数的性质[16],根据单个随机变量的概率密度函数,可得多个随机变量耦合关系下的联合概率密度函数. 建立2种随机变量情况[17]式中:F 1(y I )、F 2(y II )、f 1(y I )、f 2(y II )分别为随机变量y I 、y II 的累计概率密度函数与概率密度函数;ΩRI 、ΩRII 由鲁棒优化理论进行构建,分别为描述风电、常规电负荷随机性的不确定合集.2.3 随机变量的处理及决策以风电出力为例,利用鲁棒理论,对各时段的输出功率构建加法不确定合集:P e −s W i ,t P e W i ,t P e −u W i ,t γW i ,t式中:、Δ分别为风电场i 在t 时段的预测出力与出力偏差;Δ为出力偏差的上限;第 1 期李笑竹, 等:区域综合能源系统两阶段鲁棒博弈优化调度[J]. 浙江大学学报:工学版,2021, 55(1): 177–188.181ΓW ,t ΓW ,t δW i ,t γW i ,t P e W i ,t 为出力偏差系数;||·||∞为无穷范数;||·||1 ≤表示1范数约束对应不确定变量的空间集群效应,既在某个调度时段各风电场的出力偏差不可能同时达到最大,由此引入空间约束参数来调整不确定合集的边界. 若=||,Δ独立且服从正态分布,记期望和方差为0和σW *,利用Lindeberg-式中:Φ−1(·)为正态分布密度函数的反函数,αW 为风电置信概率.通过构造拉格朗日函数与线性对偶理论可知,考虑在t 时段的最极端情况,风电场出力达到不确定合集下限. 此时仅有一个风电场出力的偏e 同理建立用电不确定性合集,可得空间约束参数与极端功率情况,如下:P e W i ,t P e L i ,t 为了定量分析RIES 的收益风险,建立基于Copula-RCVaR 的多能流收益风险模型. 模型中,x 为上层目标的决策变量,随机变量y I 为风电出力偏差Δ,y II 为常规电负荷偏差Δ,定义系统运行时的损失函数为利润函数的负数,f c-l (x ,y )=−f 1.1,上层模型的目标函数2(min f 1.2)为式(29)的形式.3 模型的求解3.1 多目标鲸鱼优化算法鲸鱼算法(WOA )具有参数设置少、寻优性能强等特点,在求解精度和收敛速度上均优于粒子群算法PSO [18],已成功应用于大规模优化问题上.标准WOA 存在不能有效平衡全局与局部搜索能力,导致在迭代后期算法的多样性丧失,收敛能力不足的问题,如在文献[18]测试问题F2和F21上,算法在迭代最终收敛. 提出相关的改进策略,改进的鲸鱼算法(improved WOA ,IWOA )伪代码如下.算法:IWOA输入:Np (种群规模);D (维度);G (最大迭代次数);A_constant; X (初始种群)输出:x *(最优个体)1.F ←计算X 适应度;x *←从X 中选择最优个体;2.while (迭代停止条件不满足) do3. 通过式(34)、(35)更新a , A , C , l ;4. if |A |≥A_constant5. 在X 中随机选择不同5个个体(x r1, x r2, x r3, x r4, x r5);6. 通过式(36)更新X ;7. else 在X 中随机选择不同2个个体(x r1,x r2);8. 通过式(37)更新X ;end if9. 越界处理;计算F ;更新x *;end while 10. return x *式中:G iter 、G max 分别为当前迭代次数与最大迭代次数;r 为(0,1.0)的随机数;系数A 、C 均由收敛因子a 计算,随着迭代次数由2减小到0;l 为螺旋系数. 设置探索固定值A_constant ,当A ≥ A_con-stant 时执行全局搜索,反之为局部. 借助差分进化算法中个体的合作与竞争指导优化搜索,分别进行螺旋运动和直线运动,更新方式如下:多目标鲸鱼算法借鉴NSGAII 中的精英保留策略,利用外部存档保存进化过程中已经发现的非占优解. 当外部存档超出设定的最大容量时,采用拥挤熵的方式对Pareto 解集进行裁剪[19]. 该方法考虑相邻解的分布情况,能够合理反映非支配解之间的拥挤程度. 从问题的实际出发,需要得到一个满足各个目标的解,使用模糊数学的方式提取最优折中解,选择线性函数作为隶属度函数.3.2 复杂约束条件处理针对RIES 两阶段风险能量管理模型中复杂182浙 江 大 学 学 报(工学版)第 55 卷的等式与不等式约束,采用滤子技术对约束条件进行处理. 构造由目标函数与约束违反度组成的[20]式中:g i(Y)、h n(Y)分别为不等式与等式约束,m、n为对应的个数. 借助Pareto理论,在最小值问题上有如下定义.定义1 若F(Y i)≤F(Y j),G(Y i)≤G(Y j),则称滤子(F(Y i)),G(Y i))支配(F(Y j),G(Y j)).定义2 滤子集内的滤子互不支配.将上层模型中各集线器能量约束(式(12)~(14))与目标函数构造滤子对;其他约束均可以作为边界条件,直接利用元启发式算法处理. 下层模型储电侧电荷约束(18)与目标函数构造滤子对;可以转移冷热负荷、储能系统的可持续运行约束(20)、(22)、(25),采用动态可松弛约束处理方式[21]. 以储电为例,计算约束违反程度记为εESS-e,根据边界条件计算松弛度,根据松弛度确定调整量;其他约束可以作为边界条件.3.3 求解流程模型整体求解包括约束处理流程,如图2所示.图 2 优化调度模型的求解流程图Fig.2 Flow chart of solution process4 结果与讨论4.1 算例说明以修改的IEEE33节点配电网与CCHP系统耦合形成RIES,CCHP内设备及参数见表1、2. 表中,P max、P min分别为功率的上、下界,η为能效,C c为成本价格,GT为燃气轮机,WHR为余热回收装置,ABS为吸收式制冷机,ASR为电制冷机,表 1 CCHP内设备参数设置1Tab.1 Parameter setting 1 of each device in CCHP设备a i b i c i P max /kWP min /kWT on/offGT/hR eGTU/D/(kW·h−1)GT 2.15 2.210.1119040380 WHR27.0−3.300.7460000−−表 2 CCHP内设备参数设置2Tab.2 Parameter setting 2 of each device in CCHP 设备ηC c /(美元·kW−1)P max /kW GT−−−WHR−0.01674−ABS0.700.0122000 ASR 3.080.0152000 BO0.85−500第 1 期李笑竹, 等:区域综合能源系统两阶段鲁棒博弈优化调度[J]. 浙江大学学报:工学版,2021, 55(1): 177–188.183BO 为锅炉. RIES 包含3个虚拟能量厂,分别实现RIES 内电、热、冷负荷的需求响应,虚拟能量厂的相关参数如表3所示. 表中,VEP-e 、VEP-h 、VEP-c 分别表示电、热、冷的虚拟能量厂,P t 为占比,SOC 为容量,P ESS 为最大充放电功率,SOC pu 为归一化后的容量. 配电网中1为根节点,与上级电网相连,节点15接入总容量为55 MW 的风电厂群. 区域内电、冷、热负荷及风电出力预测见图3.图中,P L 为预测电荷. 电负荷的85%购自日前市场,设购买价格为0.4 kW·h/美元,电能在实时市场的交易价格与用电量有关,如图4所示,天然气购买价格为0.22 Kcf·h/美元. RIES 的电、热、冷售价见图4. 图中,C c 为价格. 风电、常规电负荷的惩罚系数为0.65、0.60 kW/美元.4.2 鲁棒决策分析鲁棒优化是在不确定变量的极端情况下系统进行的优化调度. 根据2.3节的分析,可以推出系统在所考虑的极端情况之外运行的概率:为了分析风电与负荷的置信概率与总数和系统运行在所考虑极端情况外的概率P OE 的关系,分别针对单个不确定变量与多不确定变量互相耦合的情况进行研究. 图5中,αW 、αL 分别为风电置信概率和常规电负荷置信概率,N W 、N L 分别为风电场数量和常规电负荷总数. 如图5(a )所示为单图 3 风电出力及各负荷的日前预报曲线Fig.3 Daily forecast of wind power output and load图 4 价格趋势图Fig.4 Price trend chart0.20.40.60.81.00.10.20.30.40.50.60.70.20.40.60.81.0P OEP 图 5 在极端情况外运行的概率关系Fig.5 Relation of operating outside extreme scenario表 3 虚拟能量厂相关参数设置Tab.3 Parameter setting of VEP类型参数数值VEP-e VEP-h VEP-c LSI P t20%−−LSI ξe LSI /(kW·h·美元−1)0.7−−LSII P t30%−−LSII ξe LSII /(kW·h·美元−1)0.45−−LT P t−25%20%LT P LT min ,t−00LT P LT max ,t−0.50.5LT ξLT /(kW·h·美元−1)−0.40.4ESS SOC/kW 250500500ESS P ESS /kW 100200200ESS ρ, ηc , ηd 1%, 0.9, 0.9−−ESS SOC pu0.2~0.9−−ESSξESS /(kW·h·美元−1)0.450.50.5184浙 江 大 学 学 报(工学版)第 55 卷个不确定变量(以风电为例),如图5(b )、(c )所示分别为2个不确定变量耦合. 图5(a )中,常规电负荷总数、置信概率固定分别为20、0.6;图5(b )中,风电常规电负荷总数均为15;图5(c )中,风电常规电负荷置信概率均为15.从图5(a )可以看出,不确定变量的置信概率增大,超出极端情况的概率降低;不确定变量总个数减小,该概率升高. 多个不确定变量耦合下超出极端情况概率的等高线间距增加且不等,说明该情况下不确定变量对系统的影响更加复杂.较图5(c )、(b )中小概率等高线包含区域较小,置信概率对系统超出极端情况的概率影响较明显.4.3 互动性分析基于建立的Copula-RCVaR 模型,对以下4个算例进行分析. 算例1:RIES 含虚拟冷/热/电厂;算例2:RIES 仅含虚拟热厂与虚拟冷厂;算例3:RIES 仅含虚拟电厂;算例4:RIES 完全不含虚拟能量厂,可调度仅为燃气轮机与锅炉. 设发电与用电偏差服从正态分布(预测精度为68.27%),考虑空间集群效应,总数量均为20,置信概率均为0.6. 运行结果见表4,虚拟能量厂优化方案见图6.表4中,B RIES 为RIES 利润,D VEP-e 、D VEP-h 、D VEP-c 分别为VEP-e 、VEP-h 、VEP-c 调度偏差功率,C VEP-e 、C VEP-h 、C VEP-c 分别为VEP-e 、VEP-h 、VEP-c 调度成本,S VEP-e 、S VEP-h 、S VEP-c 分别为归一化后的VEP-e 、VEP-h 、VEP-c 社会成本. 图6中,P 为功率,LSI-e 、LSII-e 分别表示迎峰电负荷和避峰电负荷,ESS-e 表示储电系统,LT-h 表示可转移热负荷,ESS-h 表示储热系统,LT-c 表示可转移冷负荷,ESS-c 表示储冷系统.从表4可以看出,随着不同类型VEP 的加入,对更多种类的可调度资源与储能装置集中管理,系统内包含的可调度资源种类增加,调度变得更加灵活,偏差随之减小,情况1(虚拟冷、热、电厂全参与的情况)下的偏差较情况3(仅有虚拟电厂参与的情况)下减小6%. 虚拟冷/热厂中包含的可控负荷主要为TL-h/TL-c ,基于该类型负荷转移前后负荷总量不变的强约束条件,使得可转移负荷数量增加,RIES 与VEP 之间的偏差大大降低. 随着可调控资源数量的增加,分摊了VEP 在调控时的经济与社会成本,各类可控资源充分全面参与调度,VEP 的经济运行成本在VEP 全参与下较仅有虚拟电厂时减少36.3%,较虚拟冷、热厂参与时分别减少17.1%、6%;社会成本相应提高,用户的用电舒适度增高;RIES 利润逐渐增加,图 6 各算例下虚拟能量厂优化方案Fig.6 Optimization plan of VEP of each case表 4 各算例下的运行结果Tab.4 Operation result of each case算例B RIES 利润/(105美元)D VEP-e /MW D VEP-h /MW D VEP-c /MW C VEP-e /(103 美元)C VEP-h /(103 美元)C VEP-c /(103 美元)S VEP-e S VEP-h S VEP-c 算例18.62 6.008.54 2.23 2.87 4.75 1.910.810.610.71算例28.53−17.20 6.17− 5.56 1.97−0.530.60算例38.50 6.36−− 3.04−−0.77−−算例48.46−−−−−−−−−第 1 期李笑竹, 等:区域综合能源系统两阶段鲁棒博弈优化调度[J]. 浙江大学学报:工学版,2021, 55(1): 177–188.185VEP 全参与下的经济成本较不含VEP 降低1.9%.从图6可以看出,在用电高峰时段(11时—13时、19时—22时),VEP 向RIES 注入能量,保证供需与电量平衡;RIES 将盈余电量以较高的实时电价,在能量交易中心通过实时市场较平稳外送至上级电网,在保证大电网稳定运行的前提下解决负荷集中地区的高峰用电需求. 在低耗电时期,VEP 向RIES 吸收能量,以满足自身区域内可控资源的运行需求. 对比图6中各算例VEP 的调度方案可知,VEP 全参与下的计划较其他2种方式更平稳,图6(a )的累积调度相对集中在[−600,600] kW ,与表4的结果吻合.4.4 运行结果敏感性分析4.4.1 不确定变量置信概率的影响 分析各不确定合集的置信概率对RIES 利润、收益损失风险的影响. 在相同风险阈值下,CVaR 置信度为0.95;不确定变量的预测精度均为68.27%;风电场、常规电负荷总数分别为20、32,不同置信概率α下的RIES 利润、收益损失风险见表5. 表中,收益损失风险为归一化后的数值. 可以看出,随着置信概率的不断减小,不确定合集区间逐渐收缩,系统所需旋转备用成本不断减小,RIES 利润随之升高;系统的收益损失风险为仅考虑系统不确定合集内的不确定性计算而来,由于不确定合集收缩,RIES 收益损失风险逐渐减小,意味着系统运行时面临的风险逐渐减小. 盲目减小置信概率,会使得系统运行在极端情况外的概率大大增加,当置信概率降至20%时,该概率为100%. 当置信概率为30%~45%时,RIES 利润增加最快,收益损失风险下降最快;当置信概率为45%~60%时,极端情况外运行概率处于可接受的低概率段.如图7所示为当α=55.5%时,上层与下层的Pareto 有效前沿. 图中,CVaR RIES 为RIES 收益风险,D B 为RIES 利润的相反数. 可以看出,利用改进的多目标鲸鱼算法得到的Pareto 解集较均匀地分布在Pareto 前沿上,具有较好的分布性. Pareto 解集中的每一点对应在该利润与收益损失风险下的RIES 及各VEP 的优化运行策略. 系统调度员可以根据实际中的不同情况,平衡RIES 风险与利润、各VEP 的偏差与成本进行决策,寻找合适的最优折中解.为了说明Pareto 最优解集为有效解,当α=55.5%时双层模型中各目标函数的收敛情况如图8所示. 图中,D VEP 为调度偏差功率,N it 为迭代次数.图 7 α=55.5% 时的 Pareto 有效前沿Fig.7 Pareto frontier for α=55.5%表 5 不同置信概率下的结果比较Tab.5 Results with different confidence probabilitiesα/%空间约束参数B RIES /(105美元)CVaR RIES P OE /%ΓW ,tΓe L ,t6019.230.28.220.982 50.0255.517.627.48.450.911 80.094514.521.68.710.784 5 1.11309.714.69.280.536 124.2320 5.98.69.530.351 3100.00100.590.899.660.351 3100.00186浙 江 大 学 学 报(工学版)第 55 卷。
关于考虑共享储能的社区综合能源系统协同优化探讨
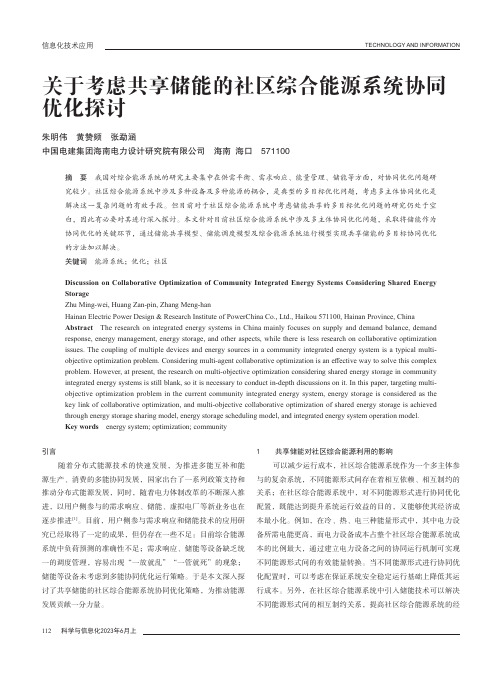
TECHNOLOGY AND INFORMATION112 科学与信息化2023年6月上关于考虑共享储能的社区综合能源系统协同优化探讨朱明伟 黄赞频 张勐涵中国电建集团海南电力设计研究院有限公司 海南 海口 571100摘 要 我国对综合能源系统的研究主要集中在供需平衡、需求响应、能量管理、储能等方面,对协同优化问题研究较少。
社区综合能源系统中涉及多种设备及多种能源的耦合,是典型的多目标优化问题,考虑多主体协同优化是解决这一复杂问题的有效手段。
但目前对于社区综合能源系统中考虑储能共享的多目标优化问题的研究仍处于空白,因此有必要对其进行深入探讨。
本文针对目前社区综合能源系统中涉及多主体协同优化问题,采取将储能作为协同优化的关键环节,通过储能共享模型、储能调度模型及综合能源系统运行模型实现共享储能的多目标协同优化的方法加以解决。
关键词 能源系统;优化;社区Discussion on Collaborative Optimization of Community Integrated Energy Systems Considering Shared Energy StorageZhu Ming-wei, Huang Zan-pin, Zhang Meng-hanHainan Electric Power Design & Research Institute of PowerChina Co., Ltd., Haikou 571100, Hainan Province, ChinaAbstract The research on integrated energy systems in China mainly focuses on supply and demand balance, demand response, energy management, energy storage, and other aspects, while there is less research on collaborative optimization issues. The coupling of multiple devices and energy sources in a community integrated energy system is a typical multi-objective optimization problem. Considering multi-agent collaborative optimization is an effective way to solve this complex problem. However, at present, the research on multi-objective optimization considering shared energy storage in community integrated energy systems is still blank, so it is necessary to conduct in-depth discussions on it. In this paper, targeting multi-objective optimization problem in the current community integrated energy system, energy storage is considered as the key link of collaborative optimization, and multi-objective collaborative optimization of shared energy storage is achieved through energy storage sharing model, energy storage scheduling model, and integrated energy system operation model.Key words energy system; optimization; community引言随着分布式能源技术的快速发展,为推进多能互补和能源生产、消费的多能协同发展,国家出台了一系列政策支持和推动分布式能源发展,同时,随着电力体制改革的不断深入推进,以用户侧参与的需求响应、储能、虚拟电厂等新业务也在逐步推进[1]。
刍议综合电力系统能量管理PMS技术

当代化工研究XChenmical I ntermediate科研开发2019 • 01刍议综合电力系统能量管理PMS 技术*张俊国赵珞(1.黑龙江科技大学黑龙江150000 2.哈尔滨工程大学黑龙江150000)摘要:随着国家之间往来增多,航海船舶的能量管理工作也逐渐受到关注。
目前,国际航运市场低迷,常规燃料对环境污染严重受燃油价格、低航速经济性、破排放等因素的影响,节约能源对整个航运业的可持续、健康发展意义重大,为了降低船舶运行总成本,需要采用 多样化的方式来对船舶能量进行管理。
达到高智慧、高效能却又低能耗、低污染,对全球环境的保护有很重大的意义。
要尽可能改善油耗、降低二氧化破排放量,朝低破足迹与绿能节能的方向前进。
在不同船况下的最佳航行姿态建议,共同拟定出有效的能源效率策略,除 了能够有效并安全地控制燃油消耗降低成本.关键词:综合电力系统;船舶;能量管理 中图分类号:T文献标识码:ADiscussion on PMS Technology of Energy Management in Integrated Power SystemZhang Junguo , Zhao Luo(1.Heilongjiang Science and Technology University , Heilongjiang , 1500002.Harbin Engineering University , Heilongjiang , 150000)Abstract'.With the increase o f e xchanges among countries, the energy management o f n avigation ships has gradually received attention.A t present, the international shipping market is in the doldrums, and conventional f uels are seriously polluting the environment. Influenced by f uel price, low speed e conomy, carbon emissions and o ther f actors, energy conservation is o f g reat s ignificance to the sustainable and h ealthy development o f t he entire shipping industry. In order to reduce the total cost o f s hip operation, it is necessary to adopt various ways to conduct the ship energy management. To achieve high intelligence, high efficiency, low energy consumption and low p ollution is o f g reat significance to the p rotection o f t he global environment. We should try our best to improve f uel consumption and reduce carbon dioxide emissions, and move f orward in the direction o f low carbon and g reen energy conservation. In order to control f uel consumption and r educe costs effectively and s afely, we should w ork out an effective energy efficiency strategy together with the best sailing advice under different ship conditions.Key words i integrated p ower system ; ships ; energy management下转第163页上接第161页[4] Biswas, S. B., P. H. Chen, and E. E. Biswas, S t r u c t u r e andF u n c t i o n of E s c h e r i c h i a -C o l i D n a b P r o t e i n - R o l e of the N -T e r m i n a l D o m a i n in H e l i c a s e Activity. B i o c h e m i s t r y , 1994. 33 (37) :p. 11307-11314.[5]Bailey, S. ,W. K. Eliason, a n d T. A. Steitz, S t r u c t u r e ofh e x a m e r i c D n a B h e l i c a s e and its c o m p l e x w i t h a d o m a i n of D n a G primase. Science, 2007. 318 (5849): p. 459-463.[6]Barcena, M., et a l.,The DnaB. D n a C complex: a s t r u c t u r e b a s e d on d i m e r s a s s e m b l e d a r o u n d an o c c l u d e d channel. E M B 0 J, 2001. 20 (6): p. 1462-8.[7]N a k a y a m a N, A r a i N, K a z i r o Y, et al. S t r u c t u r a l andf u n c t i o n a l s t u d i e s of the D n a B p r o t e i n u s i n gl imitedp r o t e olysis. C h a r a c t e r i z a t i o n of d o m a i n s for D N A -d e p e n d e n t A T P h y d r o l y s i s a n d for p r o t e i n a s s o c i a t i o n in the p r i m o s o m e [J]. J o urnal of B i o l o g i c a l Chemi s t r y , 1984, 259 (1):88-96.[8]B a i l e y S, E l i a s o n W K, S t e i t z T A. S t r u c t u r e ofh e x a m e r i c D n a B h e l i c a s e and its c o m p l e x w i t h a d o m a i n of D n a G p r i m a s e [J ]. Science, 2007, 318 (5849): 459-463.[9] S t r y c h a r s k a M S, Ariaspaloino E,L y u b i m o v A Y, etal. N u c l e o t i d e a n d p a r t n e r -p r o t e i n c o n t r o l of b a c t e r i a l r e p l i c a t i v e h e l i c a s e s t r u c t u r e a n d function. [J].M o l e c u l a r Cell, 2013, 52 (6): 844-854.[10] L o Y H, T sai K L, Sun Y J, et al.T h e c r ystals t r u c t u r e of a r e p l i c a t i v e h e x a m e r i c h e l i c a s e D n a C anditsc o m p l e x w i t h s i n g l e -s t r a nde d D N A [J ]. N u c l e i c Aci d s Research, 2009, 37 (3): 804-814.[11] Wang, G., Klein, M. G., Tokonzaba, E., Zhang, Y., Holden, L.G.,and Chen, X. S. T h e s t r u c t u r e o f a D n a B -f a m i l y r e p l i c a t i v e h e l i c a s e a n d its i n t e r a c t i o n s w i t h p r i m a s e [J ]. Nat. Struct. Mol. Biol, 2008, 15, 94-100.[12] S o u l t a n a s ,P.. L o a d i n g m e c h a n i s m s of rin g h e l i c a s e s at r e p l i c a t i o n o r i g i n s [J ]. Mol. M i c r o b i o l , 2012, 84, 6-16.[13]Biswas, S. B., Flowers, S., andBiswas-Fiss, E. E. Quantitativea n a l y s i s of n u c l e o t i d e m o d u l a t i o n of D N Ab i n d i n g b y Dna Cp r o t e i n of E s c h e r i c h i a coli [J]• Biochem. J, 2004, 379, 553-562.[14] Liu, B., W. K. Eliason, and T. A. S t e i t z.. S t r u c t u r e ofa h e l i c a s e -h e l i c a s e loader c o m p l e x r e v e a l s i n s i g h t s intothe m e c h a n i s mofb ac t e r i a lp r i m o s o m ea s s e mb l y [J ]. NatCommun, 2013, 4: p. 2495.[15] H o o d I V, B e r g e r J M. Viral h i j a c k i n g of a r e p l i c a t i v eh e l i c a s e loader and its i m p l i c a t i o n s f o r h e l i c a s e l o ading c o n t r o l a n d p h a g e r e p l i c a t i o n [J]. E l i f e ,2016,5.[16] D a v e y M J, 0r D o n n e l l M. R e p l i c a t i v e h e l i c a s e loaders:ring b r e a k e r s a n d rin g makers. [J]. C u r r e n t Biology, 2003,13 (15):R594-R596.【作者简介】陈炫志,男,成都实验外国语学校;研究方向:生物。
INTEGRATED SYSTEM FOR HEATING, COOLING AND HEAT RE
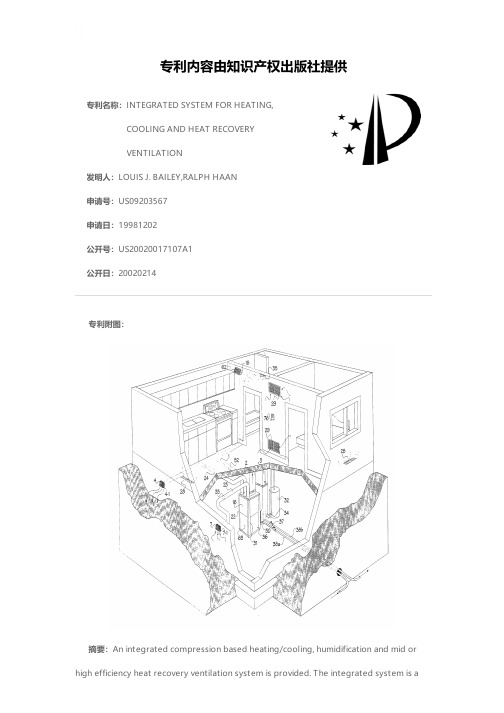
专利名称:INTEGRATED SYSTEM FOR HEATING,COOLING AND HEAT RECOVERYVENTILATION发明人:LOUIS J. BAILEY,RALPH HAAN申请号:US09203567申请日:19981202公开号:US20020017107A1公开日:20020214专利内容由知识产权出版社提供专利附图:摘要:An integrated compression based heating/cooling, humidification and mid or high efficiency heat recovery ventilation system is provided. The integrated system is acentralized apparatus that offers a very efficient method for heating/cooling with more winter and summer humidity control plus offering heat recovery ventilation in a structure having a regulated indoor temperature and specific ventilation/energy recovery rate. The heating and cooling section of the apparatus would most commonly be configured as a forced air geothermal or air-to-air heat pump. The heat recovery ventilation section of the apparatus will incorporate a heat recovery chamber complete with a primary passive cross flow heat recovery core. Plus, a secondary refrigeration based high efficiency reversible energy recovery evaporator/condenser coil. The integrated system will also incorporate a humidification system designed to operate during the winter heating season.申请人:BAILEY LOUIS J.,HAAN RALPH更多信息请下载全文后查看。
iet energy systems integration模板
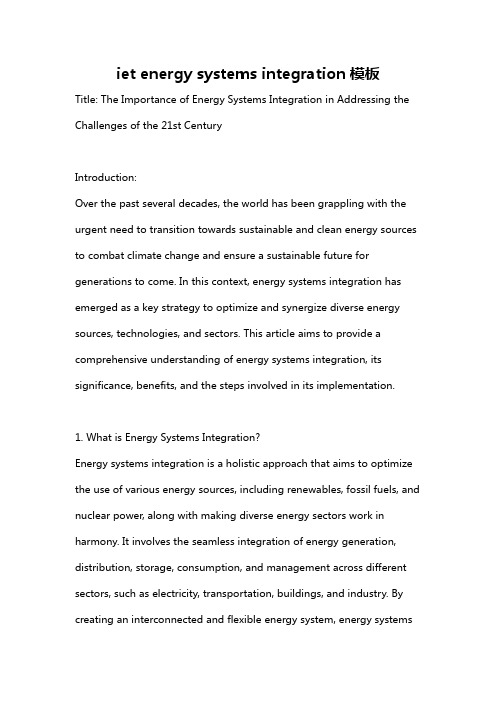
iet energy systems integration模板Title: The Importance of Energy Systems Integration in Addressing the Challenges of the 21st CenturyIntroduction:Over the past several decades, the world has been grappling with the urgent need to transition towards sustainable and clean energy sources to combat climate change and ensure a sustainable future for generations to come. In this context, energy systems integration has emerged as a key strategy to optimize and synergize diverse energy sources, technologies, and sectors. This article aims to provide a comprehensive understanding of energy systems integration, its significance, benefits, and the steps involved in its implementation.1. What is Energy Systems Integration?Energy systems integration is a holistic approach that aims to optimize the use of various energy sources, including renewables, fossil fuels, and nuclear power, along with making diverse energy sectors work in harmony. It involves the seamless integration of energy generation, distribution, storage, consumption, and management across different sectors, such as electricity, transportation, buildings, and industry. By creating an interconnected and flexible energy system, energy systemsintegration enables the efficient utilization of resources and maximizes the overall system performance.2. Significance of Energy Systems Integration:a. Decarbonization: Energy systems integration facilitates the integration of renewable energy sources into the grid, reducing the reliance on fossil fuels and ultimately lowering carbon emissions. This transition towards decarbonization is crucial for mitigating climate change and achieving a sustainable future.b. Resource Optimization: By integrating diverse energy sources, energy systems integration ensures optimal utilization of energy resources. It allows for the effective balancing of intermittent renewables with stable power sources, enables the utilization of excess renewable energy for other applications, and minimizes energy wastage.c. Resilience and Reliability: An integrated energy system enhances the resilience and reliability of power supply, reducing the risk of blackouts and disruptions. Diversifying energy sources and incorporating energy storage technologies enable a more robust and stable energy infrastructure.d. Cost Reduction: Energy systems integration helps to reduce energy costs through increased efficiency and improved energy management. By leveraging synergies among various sectors, such as combiningheating and electricity systems, cost-effective solutions can be developed.3. Steps in Energy Systems Integration:a. Mapping and Analysis: The first step involves mapping existing energy systems, identifying potential integration opportunities, and analyzing technical and economic feasibility. This includes assessing the availability of different energy sources, evaluating infrastructure requirements, and estimating the energy demands of various sectors.b. Planning and Design: The next step involves designing an integrated energy system blueprint. This includes determining the optimal energy mix, technology choices, and infrastructure requirements to meet the projected energy demand. This stage also involves considering policy frameworks, regulatory aspects, and stakeholder engagement.c. Infrastructure Development: The physical set-up of an integrated energy system requires the development of robust infrastructure, such as smart grids, energy storage facilities, and distribution networks. This includes creating interconnections and upgrading existing infrastructure to accommodate the integration of diverse energy sources.d. Policy and Regulatory Framework: Implementing energy systems integration requires a supportive policy and regulatory environment. Governments and regulators play a crucial role in providing incentives,setting standards, and establishing market mechanisms to encourage integration and create a level playing field for different energy sources and sectors.e. Monitoring and Evaluation: Continuous monitoring and evaluation of the integrated energy system's performance are essential to identify areas for improvement, optimize processes, and ensure compliance with set goals. This involves collecting data, analyzing performance indicators, and making necessary adjustments to maximize efficiency and effectiveness.Conclusion:Energy systems integration holds immense potential to transform the global energy landscape by enabling the integration and optimization of diverse energy sources, promoting decarbonization, and making energy systems more resilient and cost-effective. Governments, industries, and communities must collaborate to create supportive policy frameworks, invest in infrastructure, and leverage emerging technologies to unlock the benefits of energy systems integration. By adopting this holistic approach, we can pave the way for a sustainable and prosperous future for all.。
Clean Energy Systems

Clean Energy SystemsClean energy systems have become increasingly important in today's world as the need to reduce carbon emissions and combat climate change becomes more urgent. These systems are designed to generate energy without relying on fossil fuels, which are a major contributor to greenhouse gas emissions. While there are various types of clean energy systems, such as solar, wind, hydroelectric, and geothermal, each comes with its unique set of challenges and benefits. In this response, we will explore the significance of clean energy systems, the challenges they face, and the potential benefits they offer. One of the most significant aspects of clean energy systems is their potential to reduce the environmental impact of energy production. Fossil fuels, such as coal, oil, and natural gas, are the primary sources of energy worldwide, but their extraction and consumption release large amounts of carbon dioxide and other greenhouse gases into the atmosphere. This, in turn, contributes to global warming and climate change. Clean energy systems, on the other hand, produce energy with minimal or no emissions, making them a crucial part of efforts to mitigate climate change. Another key benefit of clean energy systems is their potential to improve public health. The burning of fossil fuels not only contributes to climate change but also releases harmful pollutants into the air, such as particulate matter, nitrogen oxides, and sulfur dioxide. These pollutants can have serious health effects, including respiratory problems, cardiovascular disease, and premature death. By transitioning to clean energy systems, we can reduce air pollution and its associated health risks, leading to a healthier population overall. In addition to the environmental and health benefits, clean energy systems also offer economic advantages. As the technology behind these systems continues to advance, the cost of implementing and maintaining them has decreased significantly. This has made clean energy more accessible and affordable for both individuals and businesses. Furthermore, the transition to clean energy has the potential to create jobs in the renewable energy sector, providing economic opportunities for communities and contributing to overall economic growth. Despite these benefits, clean energy systems also face several challenges that need to be addressed. One of the most significant challenges is the intermittency of renewable energy sources, such as solar andwind. Unlike fossil fuel power plants, which can produce a consistent amount of energy regardless of the weather, renewable energy generation is dependent on factors like sunlight and wind speed. This variability can make it difficult to integrate renewable energy into existing power grids and ensure a reliable energy supply. Another challenge is the need for significant upfront investment in clean energy infrastructure. While the long-term operational costs of clean energy systems are generally lower than those of fossil fuel-based systems, the initial capital required to build and install renewable energy technologies can be substantial. This financial barrier can make it challenging for some communities and countries to transition to clean energy, especially in the absence of supportive policies and incentives. Furthermore, the geographical limitations of certain clean energy sources, such as solar and wind, can also pose challenges.For example, not all regions receive consistent sunlight or wind patterns, makingit more difficult to harness these energy sources effectively. Additionally, the development of certain clean energy technologies, such as offshore wind farms or geothermal power plants, may face opposition from local communities or encounter regulatory hurdles, further complicating the transition to clean energy. Despite these challenges, the potential benefits of clean energy systems are toosignificant to ignore. By investing in research and development to improve the efficiency and reliability of renewable energy technologies, addressing the intermittency and geographical limitations of clean energy sources, and implementing supportive policies and incentives, we can overcome these challenges and accelerate the transition to clean energy. Doing so is crucial for mitigating climate change, improving public health, and fostering economic growth. In conclusion, clean energy systems play a vital role in addressing the environmental, health, and economic challenges associated with traditional fossil fuel-based energy production. While they offer significant benefits, such as reducing greenhouse gas emissions, improving public health, and creating economic opportunities, they also face challenges related to intermittency, upfront investment, and geographical limitations. By addressing these challenges through continued innovation, supportive policies, and strategic investments, we canunlock the full potential of clean energy systems and create a more sustainable and resilient energy future for generations to come.。
园区综合能源系统 英语

园区综合能源系统英语English:The integrated energy system in a park refers to the centralized management and utilization of multiple energy sources within the park premises to meet the various energy needs of the facilities, such as electricity, heating, cooling, and hot water. This system typically incorporates renewable energy sources like solar, wind, and geothermal to reduce reliance on traditional fossil fuels and minimize the environmental impact. By integrating different energy sources and implementing advanced energy management technologies, a park can achieve optimal energy efficiency, lower operational costs, and contribute to sustainable development. Additionally, the integrated energy system allows for better resource utilization, increased energy independence, and improved resilience to power outages or disruptions. Through the effective integration of energy systems, a park can not only enhance its operational efficiency but also promote a greener and more sustainable environment.中文翻译:园区综合能源系统是指在园区范围内集中管理和利用多种能源来源,以满足设施对电力、供暖、制冷和热水等各种能源需求。
英文 综合能源

英文综合能源
英文综合能源可以翻译为 "Integrated Energy"。
综合能源是指将各种能源进行优化组合,形成一种多能互补、协调发展的能源利用方式。
这种方式可以充分发挥各种能源的优势,提高能源利用效率,减少环境污染,实现可持续发展。
综合能源的英文翻译也可以使用 "Comprehensive Energy" 或 "Holistic Energy"。
这些翻译都强调了综合能源的全面性和整体性,涵盖了各种能源形式和利用方式,包括化石能源、可再生能源、核能等。
综合能源的发展需要依靠科技进步和政策支持。
通过研发新的能源技术和优化现有的能源系统,可以实现能源的节约、高效和环保。
同时,政府也需要制定相关的政策和标准,鼓励和推动综合能源的发展和应用。
综合能源的应用场景非常广泛,可以应用于城市、工业、交通、建筑等多个领域。
在城市中,综合能源可以应用于智能电网、分布式能源系统、多能互补系统等方面,提高城市的能源供应安全和环保水平。
在工业中,综合能源可以应用于余热回收、能源管理等方面,提高工业生产的效率和环保性能。
在交通中,综合能源可以应用于电动汽车、智能交通系统等方面,推动交通领域的绿色发展。
在建筑中,综合能源可以应用于节能建筑、太阳能利用等方面,实现建筑节能减排的目标。
总之,综合能源是未来能源发展的重要方向之一,具有广阔的应用前景和巨大的发展潜力。
通过加强科技创新和政策支持,推动综合能源的发展和应用,可以为人类创造更加美好的未来。
综合能源系统规划平台及能源规划应用研究
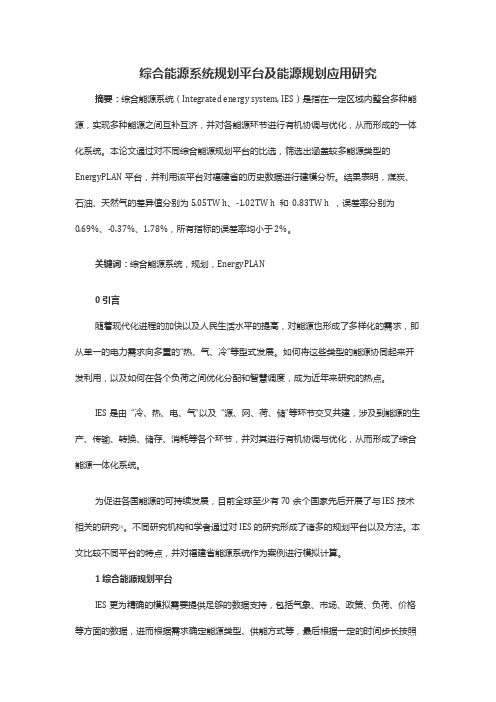
综合能源系统规划平台及能源规划应用研究摘要:综合能源系统(Integrated energy system, IES)是指在一定区域内整合多种能源,实现多种能源之间互补互济,并对各能源环节进行有机协调与优化,从而形成的一体化系统。
本论文通过对不同综合能源规划平台的比选,筛选出涵盖较多能源类型的EnergyPLAN平台,并利用该平台对福建省的历史数据进行建模分析。
结果表明,煤炭、石油、天然气的差异值分别为5.05TW h、-1.02TW h 和0.83TW h ,误差率分别为0.69%、-0.37%、1.78%,所有指标的误差率均小于2%。
关键词:综合能源系统,规划,EnergyPLAN0引言随着现代化进程的加快以及人民生活水平的提高,对能源也形成了多样化的需求,即从单一的电力需求向多重的“热、气、冷”等型式发展。
如何将这些类型的能源协同起来开发利用,以及如何在各个负荷之间优化分配和智慧调度,成为近年来研究的热点。
IES是由“冷、热、电、气”以及“源、网、荷、储”等环节交叉共建,涉及到能源的生产、传输、转换、储存、消耗等各个环节,并对其进行有机协调与优化,从而形成了综合能源一体化系统。
为促进各国能源的可持续发展,目前全球至少有70余个国家先后开展了与IES技术相关的研究[1]。
不同研究机构和学者通过对IES的研究形成了诸多的规划平台以及方法。
本文比较不同平台的特点,并对福建省能源系统作为案例进行模拟计算。
1综合能源规划平台IES更为精确的模拟需要提供足够的数据支持,包括气象、市场、政策、负荷、价格等方面的数据,进而根据需求确定能源类型、供能方式等,最后根据一定的时间步长按照表 2各能源规划软件平台支持的能源类型综合上述对比,EnergyPLAN平台涵盖较多的能源类型,可以比较完整地对一个地区的能源系统进行建模,总体上能够达到能源系统建模的要求。
为了更好的对综合能源系统进行计算,本论文选取EnergyPLAN作为建模软件平台。
服务器浸没式液冷的换热效率及节能潜力
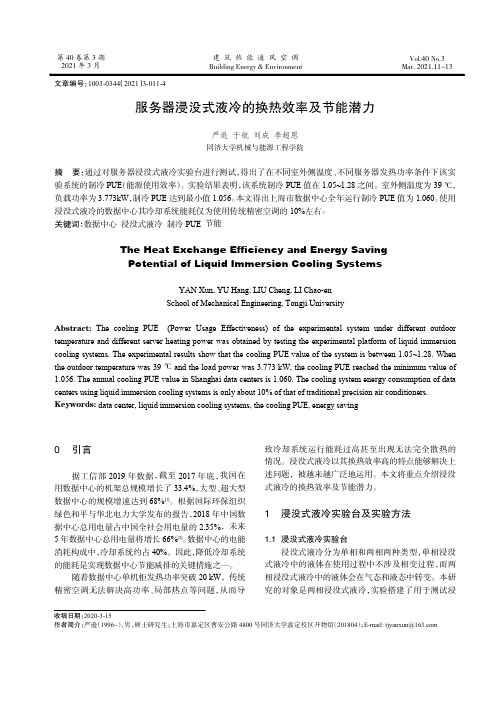
服务器浸没式液冷的换热效率及节能潜力严逊 于航 刘成 李超恩同济大学机械与能源工程学院摘 要: 通过对服务器浸没式液冷实验台进行测试, 得出了在不同室外侧温度、 不同服务器发热功率条件下该实 验系统的制冷PUE(能源使用效率)。
实验结果表明, 该系统制冷PUE 值在1.05~1.28之间。
室外侧温度为39 益, 负载功率为3.773kW ,制冷PUE 达到最小值1.056。
本文得出上海市数据中心全年运行制冷PUE 值为1.060。
使用 浸没式液冷的数据中心其冷却系统能耗仅为使用传统精密空调的10%左右。
关键词: 数据中心 浸没式液冷 制冷PUE 节能The Heat Exchange Efficiency and Energy Saving Potential of Liquid Immersion Cooling SystemsYAN Xun,YU Hang,LIU Cheng,LI Chaoen School of Mechanical Engineering,Tongji UniversityAbstract: The cooling PUE (Power Usage Effectiveness)of the experimental system under different outdoortemperature and different server heating power was obtained by testing the experimental platform of liquid immersion cooling systems.The experimental results show that the cooling PUE value of the system is between 1.05~1.28.When the outdoor temperature was 39 益and the load power was 3.773kW,the cooling PUE reached the minimum value of 1.056.The annual cooling PUE value in Shanghai data centers is 1.060.The cooling system energy consumption of data centers using liquid immersion cooling systems is only about 10%of that of traditional precision air conditioners.Keywords:data center,liquid immersion cooling systems,the cooling PUE,energy saving收稿日期: 2020315作者简介: 严逊 (1996~), 男, 硕士研究生; 上海市嘉定区曹安公路 4800号同济大学嘉定校区开物馆 (201804); Email:****************0 引言据工信部 2019 年数据,截至 2017 年底, 我国在 用数据中心的机架总规模增长了33.4%,大型、 超大型 数据中心的规模增速达到68%[1]。
考虑运行状态信息的综合能源系统图强化学习优化调度
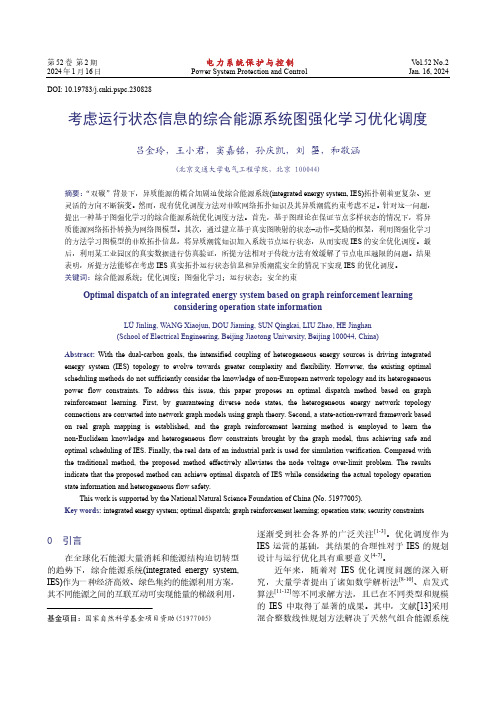
第52卷第2期电力系统保护与控制Vol.52 No.2 2024年1月16日Power System Protection and Control Jan. 16, 2024 DOI: 10.19783/ki.pspc.230828考虑运行状态信息的综合能源系统图强化学习优化调度吕金玲,王小君,窦嘉铭,孙庆凯,刘 曌,和敬涵(北京交通大学电气工程学院,北京 100044)摘要:“双碳”背景下,异质能源的耦合加剧迫使综合能源系统(integrated energy system, IES)拓扑朝着更复杂、更灵活的方向不断演变。
然而,现有优化调度方法对非欧网络拓扑知识及其异质潮流约束考虑不足。
针对这一问题,提出一种基于图强化学习的综合能源系统优化调度方法。
首先,基于图理论在保证节点多样状态的情况下,将异质能源网络拓扑转换为网络图模型。
其次,通过建立基于真实图映射的状态-动作-奖励的框架,利用图强化学习的方法学习图模型的非欧拓扑信息,将异质潮流知识加入系统节点运行状态,从而实现IES的安全优化调度。
最后,利用某工业园区的真实数据进行仿真验证,所提方法相对于传统方法有效缓解了节点电压越限的问题。
结果表明,所提方法能够在考虑IES真实拓扑运行状态信息和异质潮流安全的情况下实现IES的优化调度。
关键词:综合能源系统;优化调度;图强化学习;运行状态;安全约束Optimal dispatch of an integrated energy system based on graph reinforcement learningconsidering operation state informationLÜ Jinling, WANG Xiaojun, DOU Jiaming, SUN Qingkai, LIU Zhao, HE Jinghan(School of Electrical Engineering, Beijing Jiaotong University, Beijing 100044, China)Abstract: With the dual-carbon goals, the intensified coupling of heterogeneous energy sources is driving integrated energy system (IES) topology to evolve towards greater complexity and flexibility. However, the existing optimal scheduling methods do not sufficiently consider the knowledge of non-European network topology and its heterogeneous power flow constraints. To address this issue, this paper proposes an optimal dispatch method based on graph reinforcement learning. First, by guaranteeing diverse node states, the heterogeneous energy network topology connections are converted into network graph models using graph theory. Second, a state-action-reward framework based on real graph mapping is established, and the graph reinforcement learning method is employed to learn the non-Euclidean knowledge and heterogeneous flow constraints brought by the graph model, thus achieving safe and optimal scheduling of IES. Finally, the real data of an industrial park is used for simulation verification. Compared with the traditional method, the proposed method effectively alleviates the node voltage over-limit problem. The results indicate that the proposed method can achieve optimal dispatch of IES while considering the actual topology operation state information and heterogeneous flow safety.This work is supported by the National Natural Science Foundation of China (No. 51977005).Key words: integrated energy system; optimal dispatch; graph reinforcement learning; operation state;security constraints0 引言在全球化石能源大量消耗和能源结构迫切转型的趋势下,综合能源系统(integrated energy system, IES)作为一种经济高效、绿色集约的能源利用方案,其不同能源之间的互联互动可实现能量的梯级利用,基金项目:国家自然科学基金项目资助(51977005) 逐渐受到社会各界的广泛关注[1-3]。
集成电池热管理系统大巴空调在城市公交车上的应用
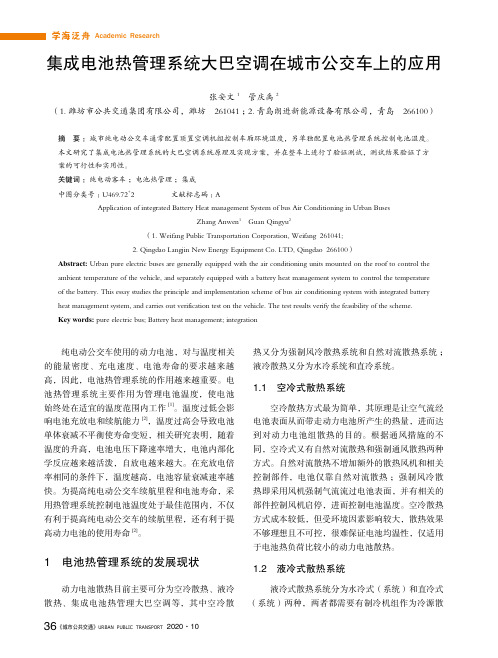
集成电池热管理系统大巴空调在城市公交车上的应用张安文1 管庆禹2(1.潍坊市公共交通集团有限公司,潍坊 261041;2.青岛朗进新能源设备有限公司,青岛 266100)摘 要:城市纯电动公交车通常配置顶置空调机组控制车厢环境温度,另单独配置电池热管理系统控制电池温度。
本文研究了集成电池热管理系统的大巴空调系统原理及实现方案,并在整车上进行了验证测试,测试结果验证了方案的可行性和实用性。
关键词:纯电动客车;电池热管理;集成中图分类号:U469.72+2 文献标志码:AApplication of integrated Battery Heat management System of bus Air Conditioning in Urban BusesZhang Anwen1 Guan Qingyu2(1. Weifang Public Transportation Corporation, Weifang 261041; 2. Qingdao Langjin New Energy Equipment Co. LTD, Qingdao 266100)Abstract: Urban pure electric buses are generally equipped with the air conditioning units mounted on the roof to control the ambient temperature of the vehicle, and separately equipped with a battery heat management system to control the temperature of the battery. This essay studies the principle and implementation scheme of bus air conditioning system with integrated battery heat management system, and carries out verification test on the vehicle. The test results verify the feasibility of the scheme.Key words: pure electric bus; Battery heat management; integration纯电动公交车使用的动力电池,对与温度相关的能量密度、充电速度、电池寿命的要求越来越高,因此,电池热管理系统的作用越来越重要。
Clean Energy Systems
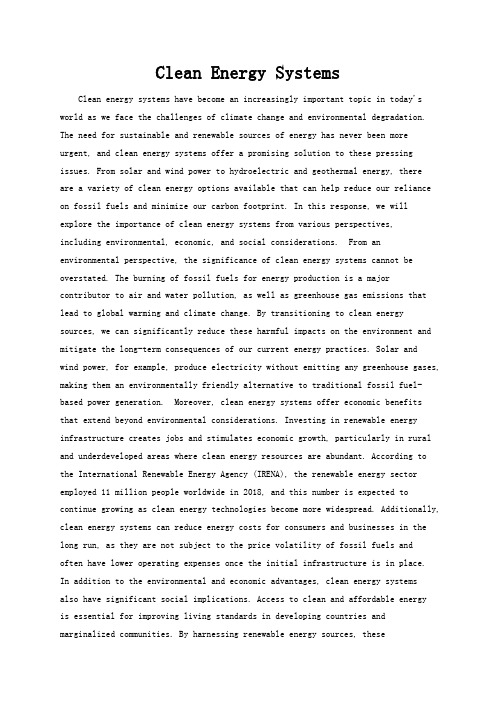
Clean Energy SystemsClean energy systems have become an increasingly important topic in today's world as we face the challenges of climate change and environmental degradation. The need for sustainable and renewable sources of energy has never been more urgent, and clean energy systems offer a promising solution to these pressing issues. From solar and wind power to hydroelectric and geothermal energy, thereare a variety of clean energy options available that can help reduce our reliance on fossil fuels and minimize our carbon footprint. In this response, we will explore the importance of clean energy systems from various perspectives,including environmental, economic, and social considerations. From an environmental perspective, the significance of clean energy systems cannot be overstated. The burning of fossil fuels for energy production is a majorcontributor to air and water pollution, as well as greenhouse gas emissions that lead to global warming and climate change. By transitioning to clean energy sources, we can significantly reduce these harmful impacts on the environment and mitigate the long-term consequences of our current energy practices. Solar andwind power, for example, produce electricity without emitting any greenhouse gases, making them an environmentally friendly alternative to traditional fossil fuel-based power generation. Moreover, clean energy systems offer economic benefitsthat extend beyond environmental considerations. Investing in renewable energy infrastructure creates jobs and stimulates economic growth, particularly in rural and underdeveloped areas where clean energy resources are abundant. According to the International Renewable Energy Agency (IRENA), the renewable energy sector employed 11 million people worldwide in 2018, and this number is expected to continue growing as clean energy technologies become more widespread. Additionally, clean energy systems can reduce energy costs for consumers and businesses in the long run, as they are not subject to the price volatility of fossil fuels andoften have lower operating expenses once the initial infrastructure is in place. In addition to the environmental and economic advantages, clean energy systemsalso have significant social implications. Access to clean and affordable energyis essential for improving living standards in developing countries and marginalized communities. By harnessing renewable energy sources, thesepopulations can gain access to electricity, heating, and cooling without further depleting natural resources or exacerbating pollution. Furthermore, clean energy technologies can enhance energy security by diversifying the energy mix and reducing dependence on imported fossil fuels, which is particularly important for countries vulnerable to geopolitical instability or fluctuating global energy markets. Despite the numerous benefits of clean energy systems, there are challenges and barriers that need to be addressed to accelerate their adoption and implementation. One of the main obstacles is the upfront cost of transitioning to clean energy, as it often requires significant investment in infrastructure and technology. Governments and private sector entities must collaborate to provide incentives, subsidies, and financing options to make clean energy more affordable and accessible to a wider range of consumers. Additionally, there is a need for continued research and development to improve the efficiency and reliability of clean energy systems, as well as to overcome technical limitations associated with energy storage and grid integration. Furthermore, the transition to clean energy requires a shift in mindset and behavior at both individual and institutional levels. Public awareness and education campaigns are essential for promoting the benefits of clean energy and dispelling misconceptions or resistance to change. It is important to engage with communities and stakeholders to ensure that clean energy projects are socially and culturally acceptable, and to address any concerns related to land use, wildlife conservation, or visual impacts associated with renewable energy infrastructure. In conclusion, clean energy systems play a crucial role in addressing the environmental, economic, and social challenges of our time. By embracing renewable energy sources and investing in clean energy technologies, we can reduce our carbon footprint, create new job opportunities, and improve access to energy for underserved populations. However, the successful transition to clean energy requires concerted efforts from policymakers, industry leaders, and the public to overcome financial, technical, and social barriers. It is imperative that we work together to accelerate the adoption of clean energy systems and pave the way for a more sustainable and resilient future for generations to come.。
Clean Energy Systems

Clean Energy SystemsClean energy systems have become increasingly important in today's world asthe need for sustainable and environmentally friendly energy sources continues to grow. With the increasing concerns about climate change and the depletion of non-renewable resources, the development and implementation of clean energy systems have become a top priority for governments, businesses, and individuals around the world. In this response, we will explore the various perspectives surroundingclean energy systems, including their benefits, challenges, and potential solutions. From an environmental perspective, clean energy systems offer significant benefits in reducing the negative impact of traditional energy sources on the planet. By harnessing renewable sources such as solar, wind, hydro, and geothermal power, clean energy systems can significantly reduce greenhouse gas emissions, air and water pollution, and habitat destruction. This is crucial in mitigating the effects of climate change and preserving the natural environmentfor future generations. The transition to clean energy also presents anopportunity to protect biodiversity and ecosystems, as well as to promote sustainable development and conservation efforts. On a societal level, clean energy systems have the potential to improve public health and safety by reducing the harmful emissions and by-products associated with fossil fuel combustion. This can lead to lower rates of respiratory illnesses, cardiovascular diseases, and premature deaths related to air pollution. Additionally, the decentralization of clean energy production can enhance energy security and resilience, particularlyin remote or underserved communities. By providing access to reliable and affordable energy, clean energy systems can also contribute to poverty alleviation, economic growth, and job creation, thus fostering social equity and empowerment. Economically, the transition to clean energy systems presents both opportunities and challenges. While the initial investment and infrastructure costs for renewable energy technologies may be high, the long-term benefits can outweigh these expenses. For instance, the declining costs of solar panels and wind turbines, coupled with government incentives and tax credits, have made clean energy more competitive with traditional energy sources. Furthermore, the shift towards clean energy can stimulate innovation, research, and technologicaladvancements, leading to new business opportunities and market growth. However,the transition may also pose challenges for industries and workers reliant onfossil fuels, necessitating a just and inclusive transition strategy to mitigate potential job losses and economic disruptions. Despite the numerous benefits of clean energy systems, several challenges and barriers hinder their widespread adoption and implementation. One of the primary obstacles is the intermittency and variability of renewable energy sources, which can pose challenges to gridstability and energy reliability. Energy storage technologies and smart grid solutions are being developed to address these issues, but further advancementsare needed to ensure a seamless integration of clean energy into existing energy systems. Additionally, the lack of supportive policies, regulatory frameworks, and investment incentives can impede the deployment of clean energy technologies and infrastructure, hindering their scalability and cost-effectiveness. In conclusion, clean energy systems offer a multitude of benefits across environmental, societal, and economic domains, making them a crucial component of a sustainable andresilient energy future. However, their widespread adoption and implementation require concerted efforts from governments, businesses, and individuals to overcome the existing challenges and barriers. By fostering collaboration, innovation, and investment in clean energy technologies, we can accelerate the transition towards a low-carbon and sustainable energy system, therebysafeguarding the planet and improving the well-being of current and future generations.。
- 1、下载文档前请自行甄别文档内容的完整性,平台不提供额外的编辑、内容补充、找答案等附加服务。
- 2、"仅部分预览"的文档,不可在线预览部分如存在完整性等问题,可反馈申请退款(可完整预览的文档不适用该条件!)。
- 3、如文档侵犯您的权益,请联系客服反馈,我们会尽快为您处理(人工客服工作时间:9:00-18:30)。
Event
CHP Summit CHP Data Workshop CHP Initiative Founders Meeting CCHP Roadmap Workshop CHP Vision Workshop CHP Analysis Workshop Combined Heat and Power – A New York Perspective BCHP Technology Program Planning Workshop Lake Michigan Regional CHP Roadmap Chicago, IL Workshop BCHP Process Workshop Northeast Regional CHP Roadmap Workshop International Symposium on Combined Heat and Power Pacific Northwest Regional CHP Challenge Workshop and Exposition BCHP Policy Workshop Upper Northeast CHP Workshop New Mexico Summit on Combined Heat and Power Texas CHP Workshop National CHP Roadmap Workshop National CHP Roadmap
What is IES ?
• IES is bringing together advanced technologies that will supply a building with: power, heating, cooling and ventilation in a single or modular package. • IES goes beyond just the package to include interconnection with the building and/or the electric grid. • IES can offer the option for a building to be self sufficient, stand alone, energy efficient, with or without grid connect and using diverse fuels like natural gas, oil or bio-fuels.
IES Program Overview
• The work at ORNL supports the U.S.DOE Distributed Energy Program in its mission.
• Goal: Increase the supply and use of clean energy resources and increase the reliability of the energy systems.
DISTRIBUTED ENERGY PEER REVIEW • DECEMBER 13-15, 2005 • ARLINGTON, VIRGINIA
Strategic Approach (Cont’d.)
• Partner with HVAC and power generation equipment industry, ESCOs, utilities, universities, state agencies and other stakeholders to develop cost effective CHP technologies and address regulatory, institutional, and market barriers. • Demonstrate and verify the benefits of expanded use of CHP in Buildings, develop and validate analysis and design tool software, document and widely disseminate results to stimulate market. • Develop advanced, “next generation” of highly efficient and cost reduced CHP equipment designed, optimized and manufactured as a “system” for broad market penetration.
Increases systems efficiency Provides energy choice Reduces CO2 emissions Conserves fuel resources Improves reliability Increases energy security Improves energy delivery utilization
DISTRIBUTED ENERGY PEER REVIEW • DECEMBER 13-15, 2005 • ARLINGTON, VIRGINIA
Integrated Energy Systems
• Known as:
– CCHP – Combined Cooling Heating & Power – BCHP – Building Cooling Heating & Power – CHPB – Cooling Heating and Power for Buildings – CHP – Combined Heat and Power – CHP - Cooling Heating and Power – Co-generation – District heating and cooling – Etc.
IES/CHP Roadmapping Events
Date
December 1, 1998 March 1, 1999 March 11-12, 1999 June 1-3, 1999 June 8-9, 1999 June 28, 1999 October 6, 1999 November 8-9, 1999 November 10-11, 1999 January 12-13, 2000 January 19-20, 2000 February 1-2, 2000 March 20-21, 2000 March 20-21, 2000 April 6, 2000 May 16, 2000 August 14-15, 2000 October 12-13, 2000 October, 2001
DISTRIBUTED ENERGY PEER REVIEW • DECEMBER 13-15, 2005 • ARLINGTON, VIRGINIA
IES - DOE-DE Strategic Plans
DISTRIBUTED ENERGY PEER REVIEW • DECEMBER 13-15, 2005 • ARLINGTON, VIRGINIA
– – – – Improve performance (efficiency) Increase reliability Reduce first (capital plus installation) cost Reduce maintenance cost
• Packaged Systems will simplify the evaluation, specification, bidding and purchasing of CHP systems. • This will enable many more architects, engineers, developers, and building owners to easily consider and use these systems.
Integrated Energy Systems Cooling, Heating & Power Overview
Bob DeVault Oak Ridge National Laboratory December 14, 2005
Energy Strongly Tied To Quality of Life
DISTRIBUTED ENERGY PEER REVIEW • DECEMBER 13-15, 2005 • ARLINGTON, VIRGINIA
Benefits of Packaged CHP Systems
• Compared to 2001’s custom engineered CHP systems, packaged systems should:
DISTRIBUTED ENERGY PEER REVIEW • DECEMBER 13-15, 2005 • ARLINGTON, VIRGINIA
Natural Gas – Important Role for Electricity
DISTRIBUTED ENERGY PEER REVIEW • DECEMBER 13-15, 2005 • ARLINGTON, VIRGINIA
• Basically they all perform similar functions
DISTRIBUTED ENERGY PEER REVIEW • DECEMBER 13-15, 2005 • ARLINGTON, VIRGINIA
Distributed Energy-IES Benefits
• Funding:
– FY04 - $9,119K – FY05 - $8,750K – Industry co-funding: $30,663,908 foቤተ መጻሕፍቲ ባይዱ packaged IES projects ($27,933,567 DOE)