Computational Design and Performance of the Fast Ocean Atmosphere Model
Geometric Modeling

Geometric ModelingGeometric modeling is a fundamental aspect of computer graphics and computer-aided design (CAD), serving as the foundation for creating and manipulatingvirtual representations of physical objects. From architectural designs to video game environments, geometric modeling plays a crucial role in various industriesand applications. This essay delves into the multifaceted nature of geometric modeling, exploring its significance, methodologies, challenges, and future directions. At its core, geometric modeling involves the representation ofobjects and their properties in a digital environment. This representation enables designers, engineers, and artists to visualize and analyze complex structures, facilitating the design process and decision-making. One of the primary methodologies in geometric modeling is the use of mathematical equations and algorithms to describe the geometry of objects. Through techniques such as parametric modeling, spline modeling, and solid modeling, practitioners can create detailed and precise digital models that accurately reflect real-world objects. Parametric modeling, for instance, allows designers to define objects using parameters such as dimensions, angles, and curves. By adjusting these parameters, they can modify the shape and size of the object dynamically, facilitating rapid prototyping and iterative design processes. Similarly, spline modeling utilizes mathematical curves to create smooth and continuous surfaces, ideal for modeling organic shapes like characters and vehicles. Solid modeling, on the other hand, focuses on representing objects as a collection of interconnected geometric primitives, enabling the simulation of physical properties such as volume and mass. Despite its versatility and utility, geometric modeling poses several challenges, ranging from computational complexity to data interoperability issues. Complex geometric operations, such as boolean operations and surface intersection calculations, can be computationally intensive, requiring efficient algorithms and computational resources. Moreover, ensuring the accuracy and consistency of geometric models across different software platforms and file formats remains a persistent challenge in the field. Interoperability standards such as the Initial Graphics Exchange Specification (IGES) and the Standard for the Exchange ofProduct model data (STEP) aim to address these challenges by providing a commonframework for exchanging geometric data between CAD systems. Beyond its practical applications, geometric modeling also intersects with artistic expression,enabling artists and animators to create visually stunning digital artworks and animations. In the realm of computer graphics, techniques such as procedural modeling and ray tracing empower artists to generate intricate scenes andrealistic lighting effects with unparalleled fidelity. Procedural modelinginvolves the use of algorithms to generate complex geometry and textures automatically, allowing artists to create vast landscapes, intricate patterns, and detailed structures with minimal manual intervention. Ray tracing, on the other hand, simulates the behavior of light in a virtual environment, enabling the rendering of photorealistic images with accurate reflections, refractions, and shadows. Looking ahead, the future of geometric modeling holds tremendous promise, driven by advancements in areas such as artificial intelligence, virtual reality, and additive manufacturing. Machine learning techniques, for instance, are revolutionizing geometric modeling by automating tedious tasks such as mesh segmentation, shape synthesis, and texture generation. By training neural networks on vast datasets of 3D models, researchers can develop algorithms that learn to understand and manipulate geometric shapes with human-like proficiency. Virtual reality technologies, meanwhile, are democratizing the creation of 3D content by providing intuitive tools for sculpting, painting, and animating virtual objectsin immersive environments. Moreover, additive manufacturing, also known as 3D printing, is expanding the possibilities of geometric modeling by enabling the fabrication of complex and customizable objects with unprecedented speed and precision. From personalized medical implants to custom-designed aerospace components, additive manufacturing is revolutionizing traditional manufacturing processes and opening new frontiers in product design and customization. By leveraging geometric modeling techniques, designers can optimize the geometries of 3D-printed objects for specific performance criteria such as strength, weight, and flexibility, unlocking new possibilities for innovation across industries. In conclusion, geometric modeling is a foundational discipline that underpins various fields ranging from engineering and architecture to entertainment and manufacturing. By leveraging mathematical principles and computational algorithms,practitioners can create, manipulate, and visualize complex geometric shapes with remarkable precision and efficiency. Despite its challenges, geometric modeling continues to evolve rapidly, driven by advancements in technology and interdisciplinary collaboration. As we look to the future, the continued integration of geometric modeling with emerging technologies promises to revolutionize how we design, create, and interact with the world around us.。
计算机领域EI和SCI收录期刊及影响因子

领域顶级国际会议,录取率 25%左右,2 年一次,中国大 陆每年论文数不超过 20 篇
模式识别,计算机 视觉,多媒体计算
15 DCC: Data Compression Conference
领域顶级国际会议,录取率很低,每年一次,目前完全 国内论文极少
数据压缩
16
ICML: International Conference on Machine Learning
网络通信领域
7
ACM MobiCom: International Conference on 无线网络领域顶级会议,录用率约为 10%,每年举行一
Mobile Computing and Networking
次。
无线网络领域
ACM SIGMETRICS: Conference on
8 Measurement and Modeling of Computer
Systems
偏重于建模和测量的重要国际会议,内容覆盖系统和网 络,录用率为 10%左右。
网络通信领域
MOBIHOC:
9
ACM International Symposium on Mobile Ad Hoc Networking and Comp
无线网络领域新兴的重要国际会议,内容侧重于 adhoc 网 络。
4:供博士生选择的相关刊物
序号 1 2 3 4 5 6 7 8 9 10 11 12 13 14 15 16 17
刊物名称(以期刊名称的拼音为序) 总被引频次
电子学报(英文版、中文版)
中文版 1676
高技术通讯(英文版、中文版) 中文版 540
计算机辅助设计与图形学学报
945
计算机工程
Hayati_et_al-2013-Microwave_and_Optical_Technology_Letters
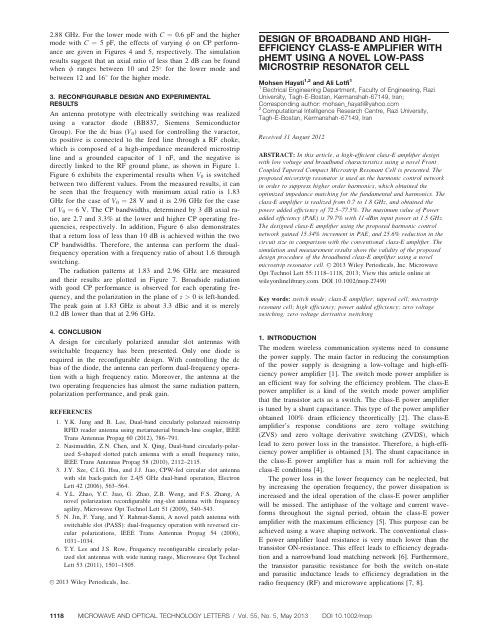
2.88GHz.For the lower mode with C¼0.6pF and the higher mode with C¼5pF,the effects of varying/on CP perform-ance are given in Figures4and5,respectively.The simulation results suggest that an axial ratio of less than2dB can be found when/ranges between10and25 for the lower mode and between12and16 for the higher mode.3.RECONFIGURABLE DESIGN AND EXPERIMENTAL RESULTSAn antenna prototype with electrically switching was realized using a varactor diode(BB837,Siemens Semiconductor Group).For the dc bias(V0)used for controlling the varactor, its positive is connected to the feed line through a RF choke, which is composed of a high-impedance meandered microstrip line and a grounded capacitor of1nF,and the negative is directly linked to the RF ground plane,as shown in Figure1. Figure6exhibits the experimental results when V0is switched between two different values.From the measured results,it can be seen that the frequency with minimum axial ratio is1.83 GHz for the case of V0¼28V and it is2.96GHz for the case of V0¼6V.The CP bandwidths,determined by3dB axial ra-tio,are2.7and3.3%at the lower and higher CP operating fre-quencies,respectively.In addition,Figure6also demonstrates that a return loss of less than10dB is achieved within the two CP bandwidths.Therefore,the antenna can perform the dual-frequency operation with a frequency ratio of about1.6through switching.The radiation patterns at1.83and2.96GHz are measured and their results are plotted in Figure7.Broadside radiation with good CP performance is observed for each operating fre-quency,and the polarization in the plane of z>0is left-handed. The peak gain at1.83GHz is about3.3dBic and it is merely 0.2dB lower than that at2.96GHz.4.CONCLUSIONA design for circularly polarized annular slot antennas with switchable frequency has been presented.Only one diode is required in the reconfigurable design.With controlling the dc bias of the diode,the antenna can perform dual-frequency opera-tion with a high frequency ratio.Moreover,the antenna at the two operating frequencies has almost the same radiation pattern, polarization performance,and peak gain.REFERENCES1.Y.K.Jung and B.Lee,Dual-band circularly polarized microstripRFID reader antenna using metamaterial branch-line coupler,IEEE Trans Antennas Propag60(2012),786–791.2.Nasimuddin,Z.N.Chen,and X.Qing,Dual-band circularly-polar-ized S-shaped slotted patch antenna with a small frequency ratio, IEEE Trans Antennas Propag58(2010),2112–2115.3.J.Y.Sze,C.I.G.Hsu,and J.J.Jiao,CPW-fed circular slot antennawith slit back-patch for2.4/5GHz dual-band operation,Electron Lett42(2006),563–564.4.Y.L.Zhao,Y.C.Jiao,G.Zhao,Z.B.Weng,and F.S.Zhang,Anovel polarization reconfigurable ring-slot antenna with frequency agility,Microwave Opt Technol Lett51(2009),540–543.5.N.Jin,F.Yang,and Y.Rahmat-Samii,A novel patch antenna withswitchable slot(PASS):dual-frequency operation with reversed cir-cular polarizations,IEEE Trans Antennas Propag54(2006), 1031–1034.6.T.Y.Lee and J.S.Row,Frequency reconfigurable circularly polar-ized slot antennas with wide tuning range,Microwave Opt Technol Lett53(2011),1501–1505.V C2013Wiley Periodicals,Inc.DESIGN OF BROADBAND AND HIGH-EFFICIENCY CLASS-E AMPLIFIER WITH pHEMT USING A NOVEL LOW-PASS MICROSTRIP RESONATOR CELLMohsen Hayati1,2and Ali Lotfi11Electrical Engineering Department,Faculty of Engineering,Razi University,Tagh-E-Bostan,Kermanshah-67149,Iran; Corresponding author:mohsen_hayati@2Computational Intelligence Research Centre,Razi University,Tagh-E-Bostan,Kermanshah-67149,IranReceived31August2012ABSTRACT:In this article,a high-efficient class-E amplifier design with low voltage and broadband characteristics using a novel Front Coupled Tapered Compact Microstrip Resonant Cell is presented.The proposed micorstrip resonator is used as the harmonic control network in order to suppress higher order harmonics,which obtained the optimized impedance matching for the fundamental and harmonics.The class-E amplifier is realized from0.7to1.8GHz,and obtained the power added efficiency of72.5–77.5%.The maximum value of Power added efficiency(PAE)is79.7%with11-dBm input power at1.5GHz. The designed class-E amplifier using the proposed harmonic control network gained15.34%increment in PAE,and25.6%reduction in the circuit size in comparison with the conventional class-E amplifier.The simulation and measurement results show the validity of the proposed design procedure of the broadband class-E amplifier using a novel microstrip resonator cell.V C2013Wiley Periodicals,Inc.Microwave Opt Technol Lett55:1118–1118,2013;View this article online at .DOI10.1002/mop.27490Key words:switch mode;class-E amplifier;tapered cell;microstrip resonant cell;high efficiency;power added efficiency;zero voltage switching;zero voltage derivative switching1.INTRODUCTIONThe modern wireless communication systems need to consume the power supply.The main factor in reducing the consumption of the power supply is designing a low-voltage and high-effi-ciency power amplifier[1].The switch mode power amplifier is an efficient way for solving the efficiency problem.The class-E power amplifier is a kind of the switch mode power amplifier that the transistor acts as a switch.The class-E power amplifier is tuned by a shunt capacitance.This type of the power amplifier obtained100%drain efficiency theoretically[2].The class-E amplifier’s response conditions are zero voltage switching (ZVS)and zero voltage derivative switching(ZVDS),which lead to zero power loss in the transistor.Therefore,a high-effi-ciency power amplifier is obtained[3].The shunt capacitance in the class-E power amplifier has a main roll for achieving the class-E conditions[4].The power loss in the lower frequency can be neglected,but by increasing the operation frequency,the power dissipation is increased and the ideal operation of the class-E power amplifier will be missed.The antiphase of the voltage and current wave-forms throughout the signal period,obtain the class-E power amplifier with the maximum efficiency[5].This purpose can be achieved using a wave shaping network.The conventional class-E power amplifier load resistance is very much lower than the transistor ON-resistance.This effect leads to efficiency degrada-tion and a narrowband load matching network[6].Furthermore, the transistor parasitic resistance for both the switch on-state and parasitic inductance leads to efficiency degradation in the radio frequency(RF)and microwave applications[7,8].The optimum operation of the class-E power amplifier and the solution to the mentioned drawbacks can be obtained using two main methods:namely active device selection and circuit configuration[9].The class-E amplifier has various configura-tions such as the cascade[10]and push–pull[11].The cascade class-E configurations can double the maximum permissible drain voltage,and the push–pull class-E configuration increases the output power and decrease the harmonic distortion with high efficiency.A new topology for the class-E amplifier is proposed as an inverse class-E amplifier,which has inductive reactance [12].The inverse class-E amplifier has higher load resistance and lower peak switch voltage in comparison with the class-E amplifier.Also,because of the abruption of the device output inductances,the value of the inductance in the load network is decreased.However,the inverse class-E amplifier can be used only for the small to medium power applications.Therefore,to solve this drawback,the power combining methods have been used[13].Although,this method results in obtaining the inverse class-E amplifier for higher power application,but the circuit configuration and the design procedure are complicated with the circuit size increment because of using two power amplifier circuits.The class-E power amplifier is a high-efficiency power am-plifier for the microwave application,which is implemented using the transmission line as the harmonic control network at the output of the amplifier circuit[14].Furthermore,instead of the RF choke(RFC)a section of the transmission line is used.The transmission line has been used in the class-E power amplifier using LDMOS[15],GaN HEMT[16–19],SiC MES-FET[20],and LDMOSFET[21]as the harmonic control net-work increasingly,because of the simplicity of its structure and high rejection of harmonics.Therefore,the class-E amplifier configuration and operation are the best candidates for the design of the amplifier for the modern microwave communica-tion systems[22,23].Consequently,designing of the load network as the harmonic control network for suppression of harmonics in order to obtain a high-efficiency power amplifier is the main challenge of the switch mode power amplifiers.The designing of the class-E power amplifiers using various microstrip structures has been proposed such as a defected ground structure[24],an asymmet-rical spur-line[25],and composite right/left-handed transmission lines[26].The narrowband load network and low efficiency remain as the main challenge to the class-E power amplifier using the conventional microstrip transmission line[27].A compact microstrip resonant cell(CMRC)is a one-dimen-sional photonic band gap incorporating the microstrip transmis-sion line,which is,first,proposed in[28].The CMRC structure exhibits high rejection of the harmonics with the compact circuit size in comparison with the conventional micorstrip transmission lines.Therefore,it is used for the linearization and efficiency in-crement of the microwave power amplifiers[29,30].The appli-cation of the conventional CMRC is limited to obtain a high-ef-ficiency switch mode amplifier,as a result of the high insertion loss in the passband and restricted stopband.The front coupled tapered CMRC(FCTCMRC)is proposed in[31]for the implan-tation of a low-passfilter with high and wide rejection in the stopband with the compact circuit size in comparison with the conventional CMRC.Therefore,it can be widely used for designing the high-efficiency and broadband switch mode power amplifier because of high and wide suppression of harmonics.In this article,the harmonic suppression of the class-E ampli-fier using a novel FCTCMRC as the harmonic controller net-work is explored.A class-E amplifier with higher efficiency at a wider bandwidth in comparison with the conventional amplifiers is achieved.The proposed class-E power amplifier is designed and simulated for a frequency of1.5GHz using the micorstrip resonator structure.The measurement results of the proposed power amplifier validate our design procedure and simulation results.2.CLASS-E AMPLIFIER FUNDAMENTAL AND DESIGN THEORY2.1.Class-E Amplifier OperationThe basic circuit configuration of the class-E amplifier and switch waveforms are shown in Figures1(a)and1(b),respec-tively.The class-E amplifier consists of the switch device,shunt capacitance,series-tuned load network L-C,and an ideal RFC. The switch-on duty ratio is assumed to be50%in designing the class-E amplifier.This value of the duty ratio leads to optimum operation of the class-E amplifier for obtaining high efficiency [32].For an ideal class-E operation,three requirements for the drain voltage and current should be met[2]:1.The rise of the voltage across the transistor at turn-offshould be delayed until the transistor is off.2.The drain voltage should be brought back to zero at thetime of the transistor turn-on.3.The slope of the drain voltage should be zero at the timeof the transistor turn-on.Therefore,the class-E power amplifier is constructed based on two conditions as ZVS and ZVDS.These conditions are as follows:v s hðÞjh¼p¼0;(1)dv s hðÞd hh¼p¼0;(2)where v s(y)is the switch voltage,and y¼x t.The quality fac-tor of the output series resonant circuit is assumed infinite. Therefore,the output current is sinusoidal asi oðhÞ¼I m sinðhþuÞ:(3)In the time interval0y<p,the switch device is in the on-state,therefore,using Kirchhoff’s current law at the switch,we havei sðhÞ¼I dc1þa sin hþuðÞðÞ:(4)This is the currentflow through the shunt capacitance in the switch-off state.Therefore,the voltage across the switchisFigure1(a)The basic circuit of the class-E amplifier.(b)The class-E switch voltage and current waveformv sðtÞ¼1C sZ ti sðt0Þdt0¼I dcx C s1þa cos x tþuðÞÀcos uðÞðÞ:(5)Applying the class-E ZVS and ZVDS conditions to Eqs.(4)and (5),the value of a and u can be obtained asa¼ffiffiffiffiffiffiffiffiffiffiffiffiffi1þp24r;(6)u¼ÀtanÀ12p8>:9>;:(7)The drain voltage waveform is shaped by the harmonics so that the drain voltage and the slope of the drain voltage is zero when the transistor is in the on-state.The reactance for all harmonics is negative and comparable in magnitude to the fundamental fre-quency load resistance.The ideal class-E amplifier requirements are difficult to meet.So,we often only tuned the second and third harmonics to get the suboptimum class-E power amplifier solution.The analysis is performed considering just the output network behavior,thus neglecting input signal required to oper-ate the active device as an ideal switch.The optimal fundamental load by the Fourier-series expan-sion analysis in[7]used for achieving the perfect class-E opera-tion can be determined asZ E;f0¼0:28x C Pe49 :(8)This impedance is inductive.On the other hand,for the ideal operation of the class-E power amplifier the impedances at the higher order harmonics are infiniteZ E;fn¼1;for n!2:(9)From(8)the nominal class-E amplifier shunt capacitance C is defined byC¼0:1836x0R:(10)In order to achieve the maximum operation frequency of the class-E amplifier,the device output capacitance should be equal to Eq.(10).The matching network for the class-E power ampli-fier using a low-pass Chebyshev-form impedance transformer is proposed in[7].Therefore,the synthesis of the load network is done using a short circuit,and open circuit stubs instead of lumped capacitors in the load network for unwanted harmonics.2.2.Design of a Class-E Amplifier Using a pHEMTAchieving the optimum load is the main factor to obtain high efficiency when designing the class-E power amplifier.On the other hand,the optimum load is varied with the operating fre-quency as in Eq.(8).Therefore,designing of the load network, which can operate in the wide frequency range,is needed for designing the class-E power amplifier with the optimum condi-tions.The maximum operation frequency of the class-E power amplifier is restricted by the shunt capacitance.The shunt capac-itance consists of the transistor output capacitance and the exter-nal capacitance.Thus,the optimum operating frequency of the class-E power amplifier is achieved by selecting a transistor with lower output capacitance.On the other hand,the power loss is caused by ON-resistance of the transistor[33].Therefore, the active device with lower ON-resistance is preferred for designing the high-efficiency class-E power amplifier.We selected an ATF-34143pHEMT because of its lower ON-resist-ance and lower shunt parasitic capacitance,which provides lower power dissipation and optimum operation frequency using external capacitance,respectively.The circuit topology of the conventional class-E amplifier is shown in Figure2(a).It is designed using the design procedure,which is presented in[2, 3].The value of elements for an ideal class-E power amplifier is tabulated in Table1.In the design of the class-E power ampli-fier,it is assumed that the value of the DC-feed is infinitive,but in real implementation this value isfinite,and we used the half wavelength microstrip transmission line for the DC-feed.In the conventional class-E amplifier,using lumped elements, the second harmonic is located within the pass band.Therefore, the bandwidth is limited to one octave.In order to solve this drawback,one way is designing a multiple matching network for various bands and using switching element.This way leads to complexity of the amplifier circuit and degradation of the efficiency.The use of the micorstrip transmission line is a low-cost and simple way for designing the class-E amplifier with wide band and high-efficiency characteristics.We used the design proce-dure in Section2.1and designed the matching network for the amplifier as shown in Figure2(b).The values of the transmis-sion lines dimensions are given in Table2.The class-E ampli-fier is designed on RT/Duroid5880,a substrate with dielectric constant of2.2,height of15l l,and loss tangent of0.0009.Figure2Idealized class-E power amplifier:(a)lumped elements and(b)transmission lineTABLE1Element Design for the Nominal Class-E AmplifierC i1(pF)C i2(pF)C o1(pF)C o2(pF)C e(pF)C g1(pF)C g2(pF)C d1(pF)C d2(pF)L i1(nH)L o1(nH)L o2(nH) Theoretical10010010010 4.2221000.50.2312 4.7 3.33.FRONT COUPLED TAPERED CMRC CHARACTERISTICSA novel FCTCMRC is proposed in [31],for the first time,which is used to synthesize a low-pass filter with high and wide rejec-tion in the stopband.This microstrip structure exhibits bandstop characteristics and slow wave effects,which are used in the stopband extension and the circuit size reduction,respectively.The schematic and equivalent circuit of the resonator is shown in Figures 3(a)and 3(b),respectively.The proposed FCTCMRC has symmetrical topology.Therefore,the even–odd mode [34]can be used to simplify the analysis as shown in Figures 3(c)and 3(d).Consequently,theresonant condition for the odd-mode in Figure 3(c)is obtained by equating the input admittance Y o in of the proposed resonator to zero yields:Z 112x C 1ÀZ 1tan h 1 ÀZ 2tan h 2Z 1þtan h 12x C 1¼0:(11)Using the similar procedure,the even-mode resonant frequencies areobtained by equating the even admittance Y e in to zero as follows:Z 2tan h 1þZ 1tan h 2¼0:(12)The transmission zeros of the equivalent circuit for the proposed FCTCMRC,which is shown in Figure 3(a),is obtained whenY o in ¼Y ein asZ 2sin 2h 2þZ 1sin 2h 1¼cos 2h 1x C 1:(13)Therefore,the resonator characteristics for tuning transmission zeroes in the stopband can be achieved by the length and width of the tapered cells as shown in Figures 4(a)and 4(b).The pro-posed structure is optimized by an EM-simulator (ADS).The obtained dimensions are as follows:L t1¼2:58;L 2¼1:94;L 3¼2:7;W t1¼2:71;W t2¼5:6;W 1¼0:1;W 2¼0:56;L 3¼0:75;L f ¼2:36;W f ¼0:25all are in millimeter ðmm Þ:TABLE 2The Value of the Conventional Transmission Line for the class-E AmplifierTL 1TL 2TL b1TL 3TL 4TL 5TL b2Width (mm) 4.730.940.620.71 1.24 4.210.72Length (mm) 6.319.7262.3137.2318.4264.3Figure 3(a)Schematic of the proposed resonator.(b)Equivalent cir-cuit.(c)Odd-mode.(d)EvenmodeFigure 4(a)Changing of the transmission zeros with the width of tapered cell W t1.(b)Changing of the transmission zeros with the length of tapered cell L t .(c)Simulation and measurement results of the proposed harmonic control network.(d)Simulation input impedance of the FCTCMRCThe proposed FCTCMRC is fabricated,and the measurement is performed using an Agilent N5230A Network Analyzer.The simulation and measurement results of the proposed FCTCMRC are shown in Figure 4(c).As it is shown,it has an attenuation level À43and À33.1dB at 3.0and 4.5GHz,respectively.Therefore,the high suppression for the second and third har-monics is obtained.The insertion loss from DC to 2.39GHz is lower than À0.1dB.The simulation of the input impedance of the proposed CMRC for the fundamental and harmonics is shown in Figure 4(d).As it is observed,the harmonic impedan-ces are relatively open in comparison with the fundamental im-pedance.Consequently,it can be used as the matching network with high performance and low circuit complexity.4.CIRCUIT DESIGN AND IMPLEMENTATIONThe highly efficient and compact size class-E amplifier is designed and implemented for a 1.5-GHz band using an ATF-34143pHEMT.The proposed circuit is simulated using an Agi-lent’s Advanced Design System (ADS),and fabricated on an RT/Duroid 5880substrate.The active device is biased at V d ¼3V and V g ¼À0.7V.The FCTCMRC is used as the harmonic control network (HCN)at the output of the active device.The proposed HCN absorbed the parasitic reactance and capacitance of the active device.Therefore,it does not need to any lumped elements in series or parallel with the transistor to compensate the parasitic elements.The circuit schematic diagram of the designed class-E amplifier is shown in Figure 5(a).Moreover,the photograph of the fabricated circuit is shown in Figure 5(b).The RFC is realized using the microstrip transmission line (TLb2)with the quarter wavelength at a frequency of 1.5GHz.The input matching elements consist of two series and parallel open stubs.The dimensions of the tapered cells and transmission lines in the HCN are tuned in order to optimize harmonic termi-nation in the implemented amplifier circuit.The design and implementation of the output matching networks using the FCTCMRC as low-pass topology has been done from 0.7to 1.8GHz.The voltage and current waveforms of the designed class-E amplifier are shown in Figure 5(c).The switch is open for the time interval,0.2–0.4ns and the current through it is near zero.The switch is closed during the time interval 0.6–0.8ns,and the voltage across it is near to zero.The class-E ZVS and ZVDS conditions in the switch turn-off state are obtained.Therefore,the high-efficiency class-E amplifier is achieved.The input signal is generated using an Agilent E4433B signal generator,and the measurement is done by an E4440A PSA se-ries spectrum analyzer.The simulated and measured output power and gain for P in ¼11dBm (input power)are shown in Figure 6(a).The maximum output power at 1.5GHz with P in ¼11dBm is 25.3dBm,and the related gain is 14.3dB.The con-ventional class-E amplifier without CMRC has an output power of 18.5dBm and gain of 7.5dB.The class-E amplifier using CMRC has 36.7%output power improvement in comparison with the one without CMRC.The simulation and measurement results for the PAE at P in ¼11dBm (input power)is shown as a function of the operating frequency in Figure 6(b).The highest value of PAE at a fre-quency of 1.5GHz was 79.7%.The value of the PAE is 69.1%for the conventional class-E amplifier without CMRC.There-fore,the proposed class-E amplifier using the novel CMRC has 15.34%PAE improvement in comparison with the one without CMRC.The output power of the conventional class-E amplifier is decreased as the operating frequency is increased.As shown in Figure 6(a),this decrement is considerable when the operating frequency is more than 1.2GHz.Therefore,the conventional class-E amplifier has a drawback for the broadband applications.The designed class-E amplifier has 25.6%circuit size reduction in comparison with the conventional class-E amplifier.5.CONCLUSIONThe class-E amplifier with high efficiency and broadband char-acteristics has been designed and implemented.A novel and simple load-matching technique for the low-voltage microwave class-E amplifier using a front-coupled taperedcompactFigure 5The pHEMT class-E amplifier.(a)Circuit configuration.(b)A photograph of fabricated amplifier.(c)Simulated switch voltage and current waveforms.[Color figure can be viewed in the online issue,which is available at ]microcstrip resonant cell has been presented.The proposed am-plifier achieved an output power of 25.3dBm,a power added efficiency of 79.7%,and a gain of 7.5dB at input power of 11dBm.It has high-efficiency performance over a significant band-width form 0.7to 1.8GHz (88%).The proposed compact micro-strip resonant cell as the harmonic control network exhibited 15.34%improvement in PAE and 25.6%reduction in the circuit size in comparison with the conventional class-E amplifier.The extremely low insertion loss at the fundamental frequency and size reduction characteristics can be used in the design of the class-E amplifier with higher output power and smaller size,which are required in the broadband application.REFERENCES1.S.C.Cripps,Advanced techniques in RF power amplifiers design,Artech House,Norwood,MA,2002.2.N.O.Sokal and A.D.Sokal,Class E—A new class of high-effi-ciency tuned single-ended switching power amplifiers,IEEE J Sol-id-State Circuits 10(1975),168–176.3.F.H.Raab,Idealized operation of the class E tuned power ampli-fier,IEEE Trans Circuits Syst 25(1977),725–735.4.R.E.Zulinski and J.W.Steadman,Class E power amplifiers and frequency multipliers with finite DC-feed inductance,IEEE Trans Circuits Syst 34(1987),1074–1087.5.R.Negra,F.M.Ghannouchi,and W.Bachtold,Study and design optimization of multi-harmonic transmission-line load networks for class-E and class-F K-band MMIC power amplifiers,IEEE Trans Microwave Theory Tech 55(2007),1390–1397.6.K.L.R.Mertens and M.S.J.Steyaert,A 700-MHz 1-W fully differ-ential CMOS class-E power amplifier,IEEE J Solid-State Circuits 37(2002),137–141.7.T.B.Mader and Z.B.Popovic,The transmission line high-effi-ciency class-E amplifier,IEEE Microwave Guided Wave Lett 5(1995),290–292.8.T.Suetsugu and M.K.Kazimierczuk,Design procedure for lossless voltage-clamped class E amplifier with a transformer and a diode,IEEE Trans Power Electron 20(2005),56–64.9.H.J €a ger,A.V.Grebennikov,E.P.Heaney,and R.Weigel,Broad-band high-efficiency monolithic In-GaP/GaAs HBT power ampli-fiers for wireless applications,Int J RF Microwave Comput Aided Eng 13(2003),496–510.10.A.Mazzanti,rcher,R.Brama,and F.Svelto,Analysis of reli-ability and power efficiency in cascode class-E PAs,IEEE J Solid--State Circuits 41(2006),1222–1229.11.S.C.Wong and C.K.Tse,Design of symmetrical class-E poweramplifiers for very low harmonic-content applications,IEEE Trans Circuits Syst I,Reg Papers 52(2005),1684–1690.12.T.Mury and V.F.Fusco,Inverse class-E amplifier with transmis-sion line harmonic suppression,IEEE Trans Circuits Syst I,Reg.Papers 54(2007),1555–1561.13.T.Mury and V.F.Fusco,Power combining techniques into unbal-anced loads for class-e and inverse class-e amplifiers,IET Micro-wave Antennas Propag 2(2008),529–537.14.A.J.Wilkinson and J.K.A.Everard,Transmission-line load-networktopology for class-E power amplifiers,IEEE Trans Microwave Theory Tech 49(2001),1202–1210.15.J.Lee,S.Kim,J.Nam,J.Kim,I.Kim,and B.Kim,Highly effi-cient LDMOS power amplifier based on class-E topology,Micro-wave Optical Technol Lett 48(2006),789–791.16.Y.-S.Lee and Y.-H.Jeong,A high-efficiency class-E GaN HEMTpower amplifier for WCDMA applications,IEEE Microwave Wire-less Compon Lett 17(2007),622–624.17.H.G.Bae,R.Negra,S.Boumaiza,and F.M.Ghannouchi,High-ef-ficiency GaN class-E power amplifier with compact harmonic-sup-pression network,Proc 37th Europ Microwave Conf,2007,pp.1093–1096.18.Y.-S.Lee,M.-W.Lee,and Y.-H.Jeong,A 1-GHz GaN HEMTbased class-E power amplifier with 80%efficiency,Microwave Opt Technol Lett 50(2008),2989–2992.19.Y.-S.Lee,M.-W.Lee,and Y.-H.Jeong,A 40-W balanced GaNHEMT class-E power amplifier with 71%efficiency for WCDMA base station,Microwave Opt Technol Lett 51(2009),842–845.20.Y.S.Lee and Y.H.Jeong,A high-efficiency class-E power ampli-fier using SiC MESFET,Microwave Opt Technol Lett 49(2007),1447–1449.21.J.-H.Van,M.-S.Kim,S.-C.Jung,H.-C.Park,G.Ahn,C.-S.Park,B.-S.Kim,and Y.Yang,A high-frequency and high-power quasi-class-E amplifier design using a finite bias feed inductor,Micro-wave Opt Technol Lett 49(2007),1114–1118.22.R.Beltran,F.H.Raab,and A.Velazquez,High-efficiency out phas-ing transmitter using class-E power amplifiers and asymmetric combining,Microwave Opt Technol Lett 51(2009),2959–2963.23.C.Park,Y.Kim,H.Kim,and S.Hong,Fully integrated 1.9-GHzCMOS power amplifier for polar transmitter applications,Micro-wave Opt Technol Lett 48(2006),2053–2056.24.Y.C.Jeong,S.-G.Jeong,J.S.Lim,and S.W.Nam,A new methodto suppress harmonics using k /4bias line combined by defected ground structure in power amplifiers,IEEE Microwave Wireless Compon Lett 13(2003),538–540.25.L.Wang,W.Chen,P.Wang,X.Xue,J.Dong,and Z.Feng,Design of asymmetrical spur-line filter for a high power sic MES-FET class-E power amplifier,Microwave Opt Technol Lett 52(2010),1650–1652.26.M.Thian and V.Fusco,Design strategies for dual-band class-Epower amplifier using composite right/left-handed transmission lines,Microwave Opt Technol Lett 49(2007),2784–2788.27.Y.Qin,S.Gao,A.Sambell,and E.Korolkiewicz,Design of low-cost broadband class-e power amplifier using low-voltage supply,Microwave Opt Technol Lett 44(2005),103–106.28.Q.Xue,K.M.Shum,and C.H.Chan,Novel 1-D microstrip PBGcells,IEEE Microwave Wireless Comp Lett 10(2000),403–405.29.T.Yin,Q.Xue,and C.H.Chan,Amplifier linearization using com-pact microstrip resonant cell-theory and experiment,IEEE Trans Microwave Theory Tech 52(2004),927–934.Figure 6Comparison of the conventional amplifier simulation with the simulated and measured results of the proposed amplifier.(a)Output power and gain.(b)Power added efficiency (PAE %)。
Computational Fluid Dynamics

Computational Fluid Dynamics Computational Fluid Dynamics (CFD) is a branch of fluid mechanics that uses numerical analysis and data structures to solve and analyze problems that involve fluid flows. It has become an essential tool in various industries, including aerospace, automotive, and environmental engineering. CFD allows engineers and scientists to simulate the behavior of fluids in complex systems, such as air flow over an aircraft wing or water flow in a river, without the need for costly and time-consuming physical experiments. One of the key advantages of CFD is itsability to provide detailed insights into fluid flow phenomena that are difficult or impossible to observe experimentally. This is particularly useful in the design and optimization of engineering systems, where understanding the behavior offluids is crucial. For example, CFD can be used to predict the performance of a new aircraft design, optimize the cooling system of a car engine, or analyze the dispersion of pollutants in the atmosphere. By simulating these scenarios, engineers can make informed decisions and improve the efficiency and safety oftheir designs. However, CFD is not without its challenges. One of the main issues is the complexity and computational cost of simulating fluid flows accurately.Fluid dynamics is a highly nonlinear and chaotic phenomenon, and simulating it requires solving complex mathematical equations that describe the behavior of fluids. This often involves dividing the fluid domain into a large number of smaller elements, or "cells," and solving the equations for each cell. As a result, CFD simulations can be computationally intensive and time-consuming, requiringhigh-performance computing resources and specialized software. Another challengeis the accuracy and reliability of CFD simulations. While CFD has made significant advancements in recent years, it is still a numerical approximation of the real physical phenomena. The accuracy of CFD simulations depends on various factors, such as the quality of the mesh (the division of the fluid domain into cells), the choice of numerical methods, and the assumptions and simplifications made in the simulation. Engineers and scientists must carefully validate and verify their CFD simulations against experimental data to ensure their reliability and trustworthiness. Furthermore, CFD requires a deep understanding of fluid mechanics, numerical methods, and computer programming. Engineers and scientistsmust be well-versed in the underlying physics of fluid flows, as well as the mathematical and computational techniques used in CFD. This often requires extensive training and expertise, which can be a barrier for individuals and organizations looking to adopt CFD in their work. Despite these challenges, the potential benefits of CFD make it a valuable tool for engineers and scientists. By providing detailed insights into fluid flow phenomena, CFD enables the design and optimization of engineering systems with improved efficiency and performance. As computing power and simulation techniques continue to advance, CFD is expected to play an increasingly important role in engineering and scientific research, shaping the way we understand and manipulate fluid flows in the world around us.。
介绍计算机组成原理这门学科的英语作文

介绍计算机组成原理这门学科的英语作文Computer organization and design is a fundamental subject in the field of computer science. 计算机组成原理和设计是计算机科学领域的一门基础课程。
It focuses on the understanding and design of computer systems at the hardware level, and plays a crucial role in shaping the way modern computing systems work. 它关注的是在硬件层面上理解和设计计算机系统,并且在塑造现代计算系统的工作方式上起着至关重要的作用。
At its core, computer organization and design delves into the architecture and functionality of computer hardware components such as the CPU, memory, and I/O devices. 在其核心,计算机组成原理和设计深入研究了计算机硬件组件的体系结构和功能,如CPU、内存和I/O 设备。
It aims to provide a comprehensive understanding of how these components work together to execute instructions, process data, and perform various computational tasks. 它旨在提供对这些组件如何一起执行指令、处理数据和执行各种计算任务的全面理解。
Furthermore, the study of computer organization and design often involves exploring the principles of digital logic and circuit design. 此外,计算机组成原理和设计的研究通常涉及探索数字逻辑和电路设计的原理。
混流式喷水推进器的性能试验与数值计算
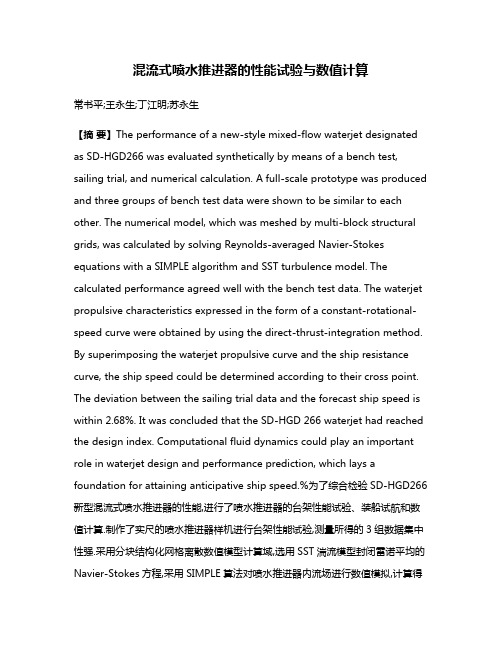
混流式喷水推进器的性能试验与数值计算常书平;王永生;丁江明;苏永生【摘要】The performance of a new-style mixed-flow waterjet designated as SD-HGD266 was evaluated synthetically by means of a bench test, sailing trial, and numerical calculation. A full-scale prototype was produced and three groups of bench test data were shown to be similar to each other. The numerical model, which was meshed by multi-block structural grids, was calculated by solving Reynolds-averaged Navier-Stokes equations with a SIMPLE algorithm and SST turbulence model. The calculated performance agreed well with the bench test data. The waterjet propulsive characteristics expressed in the form of a constant-rotational-speed curve were obtained by using the direct-thrust-integration method. By superimposing the waterjet propulsive curve and the ship resistance curve, the ship speed could be determined according to their cross point. The deviation between the sailing trial data and the forecast ship speed is within 2.68%. It was concluded that the SD-HGD 266 waterjet had reached the design index. Computational fluid dynamics could play an important role in waterjet design and performance prediction, which lays a foundation for attaining anticipative ship speed.%为了综合检验SD-HGD266新型混流式喷水推进器的性能,进行了喷水推进器的台架性能试验、装船试航和数值计算.制作了实尺的喷水推进器样机进行台架性能试验,测量所得的3组数据集中性强.采用分块结构化网格离散数值模型计算域,选用SST湍流模型封闭雷诺平均的Navier-Stokes方程,采用SIMPLE算法对喷水推进器内流场进行数值模拟,计算得到的台架性能曲线与试验结果吻合较好.采用壁面积分法获取了装船喷水推进器的等转速推进特性曲线,通过与船阻力曲线叠加求交点进行航速预报,航速预报值与实船试航值最大偏差为2.68%.结果表明,该型喷水推进器达到了设计要求,数值计算可在喷水推进器的水力设计与性能分析中发挥重要作用,为喷水推进船最终达到预期航速奠定基础.【期刊名称】《哈尔滨工程大学学报》【年(卷),期】2012(033)005【总页数】5页(P660-664)【关键词】喷水推进;台架试验;试航;数值计算;性能;试验【作者】常书平;王永生;丁江明;苏永生【作者单位】海军工程大学船舶与动力学院,湖北武汉430033;海军工程大学船舶与动力学院,湖北武汉430033;海军工程大学船舶与动力学院,湖北武汉430033;海军工程大学船舶与动力学院,湖北武汉430033【正文语种】中文【中图分类】U664.34近年来,我国先后从Rolls-Royce公司、MJP公司、Alamarin公司和Hamilton 公司引进了多型混流式喷水推进器用于高性能或特殊用途的舰船上.混流式喷水推进器具有轴向长度短、功率密度大、过流能力强、汽蚀性能好等特点,设计难度较高[1-3].目前,国内自主成功研发混流式喷水推进器的案例还很少.作者针对“飞鹰一号”快艇的快速性需求设计了一新型混流式喷水推进器,命名为SD-HGD266.本文将采用试验研究和数值模拟两种手段,综合检验该型喷水推进器是否达到了设计要求.1 喷水推进器的主要参数SD-HGD266型混流式喷水推进器的设计参数为:比转速ns=449,流量系数KQ=0.78,扬程系数KH=0.325,功率系数KP=2.859.各无量纲参数的定义为式中:Q是流量,m3/s;n是转速,r/s;D是叶轮直径,m;H是扬程,m;P是功率,kW.SD-HGD266型混流式喷水推进器的主要结构参数为:叶轮进口直径D1=266 mm,泵壳最大直径Dmax=334 mm,喷口直径Dj=155 mm,叶轮叶片数z1=4,整流器叶片数z2=7.图1 SD-HGD266喷水推进器三维造型Fig.1 3D modeling of SD-HGD266 waterjet2 喷水推进器的台架试验制作了实尺喷水推进器样机,在清水试验台上进行性能测试,试验管路布置如图2.进水流道底座作为进水流道与船底的过渡段,是为了更好地适应实船航行时的来流条件,台架试验时没有将其包括在内.另外,由于样机的喷口没用法兰盘结构而不便与试验管路连接,台架试验时采用了等外径的专用整流器.图2 喷水推进器试验管路布置Fig.2 Configuration of waterjet bench台架性能试验按以下步骤进行:1)电机启动前,出口阀闸关闭.2)电机启动后,出口阀闸开到最大.待管路内流动稳定后,从最大流量逐渐关小阀闸.每个流量点运行5 min待流动稳定后,记录进口压力、出口压力、电压、电流和转速等数据.3)从小流量点逐渐开大阀闸.每个流量点运行5 min待流动稳定后记录数据.4)流量从大到小和从小到大各测量15个流量点,所有点覆盖高效区.待前一组试验完毕,停车4 h后再进行下一组试验,共进行3组试验.扬程和功率计算公式分别为:式中:Pout和Pin分别是出口和进口平均压力;Vout和Vin分别是出口和进口的平均速度;Δz是出口面至进口面中心轴线的垂直距离;U是电压;I是电流; cos φ是功率因数;η是电机效率.从图3可得各组试验数据趋势一致、集中性强,这说明试验具有较好的可重复性,增强了试验结果的可信度.图3 喷水推进器台架试验结果Fig.3 Bench test results of waterjet3 台架喷水推进器的性能计算3.1 数值模型采用SST湍流模型[4]封闭RANS方程来求解喷水推进器内部三维流场.连续性方程[5]和动量方程分别为:式中:fi为体积力;p为作用在流体上的压力;ρ为水密度;μ为水的分子粘性系数;μt为湍流动力粘性系数.采用分块六面体结构化网格对各部件进行离散,如图4.叶轮和整流器分别采用了H型和J型拓扑结构,叶片周围嵌入O型网格进行加密,叶顶间隙采用了数层独立的H型网格,进水流道及旋转轴附近网格作加密处理以满足湍流模型对y+的要求,网格总数为2.27×104.图4 各部件网格Fig.4 Mesh of every component采用基于中心节点控制的有限体积法离散控制方程,基于SIMPLEC算法求解速压耦合方程.进口边界给定流量,出口边界设置为环境压力.旋转叶轮和静止部件之间的耦合采用多重参考坐标系(MRF)[6]模型,叶轮轮毂和叶轮叶片设置为相对静止无滑移壁面,其他壁面设为绝对静止无滑移壁面.3.2 计算结果分析将计算结果与3组试验数据的平均值比较如表1和图5,分析可得:1)在设计点计算值与试验值吻合良好,扬程、功率和效率的误差分别为0.1%、4.6%和1.78%.2)计算所得性能曲线与试验曲线趋势一致.其中,扬程最大误差小于8%,功率最大误差小于5%.3)试验值最高效率点KQ=0.766、η=0.847,计算值最高效率点KQ=0.794、η=0.830,两者偏差较小.表1 设计点的试验值与计算值Table 1 Comparison of test data and calculated data at the design condition参数KQ KH KP η试验参数0.780 0.291 2.635 0.845计算参数0.780 0.291 2.757 0.830图5 台架试验值与计算值对比Fig.5 Comparison of bench test data and calculated data图6 真实整流器的性能曲线Fig.6 Waterjet performances of with the real diffuser在验证了数值方法可信性的基础上,建立了真实整流器的数值模型.将出口取压点位置移到喷口处,以与设计参数中扬程H的定义一致.真实喷水推进器的性能计算曲线如图6,分析可得:设计点的计算值KH=0.324,KP=2.838,η=0.875,达到了设计要求;在设计点左右较宽流量范围内(0.9KQ~1.15KQ),效率都达到0.85以上,这对喷水推进器运行的稳定性和经济性十分有利;该喷水推进器的设计点基本上就是最高效率点,说明设计点把握较准确.4 装船喷水推进器性能计算与验证实船喷水推进器工作时会受船体边界层的影响,进入进水流道的水流是不均匀的.选取计算域时应将流道进水口外船体下方的流体也包括在内[7].“飞鹰一号”快艇装配的2台SD-HGD266喷水推进器关于艇体中剖面对称,为了节省计算资源,只建立了一台喷水推进器和一半船底的流场计算域如图7.船体中剖面设为对称面边界条件,船底流场控制体进流面设为速度进口,喷口压力和船底水出流压力设为环境压力.图7 实船喷水推进器数值计算域Fig.7 Computational region of the installed waterjet根据数值计算结果可得到流场中各点的速度、压力等物理量,通过积分求取出喷水推进器各壁面上的压力和粘性力,将各个面上力相加即得到喷水推进器产生的总推力,这就是壁面积分法[8-9].由于喷水推进器与船体之间存在相互作用,使得喷水推进器净推力Tnet与裸船阻力RBH有如下关系[10]:式中:t为推力减额[11].将计算得到的喷水推进器的等转速推力特性曲线和“飞鹰一号”快艇的阻力特性曲线叠加到一起,如图8.各个交点对应的航速就是该艇在相应的喷水推进器转速条件下所能达到的航速,如表3.分析可得:该艇达到了设计航速要求(37 kn);各转速条件下,数值预报的航速与实际试航值吻合较好,最大偏差约为2.68%.表2 喷水推进船的航速预报值与实船航行值比较Table 2 Speed comparison between the forecast results and the real sailing data主机转速试航航速/knCFD航速/kn 偏差37.3 38.3 2.68 n2=0.93 ndesign 35.1 35.2 0.29 n3=0.85 ndesign /% n1=ndesign 29.7 29.8 0.34图8 喷水推进船的航速预报Fig.8 Speed forecast of waterjet ship喷水推进船航速预报偏差的原因可能有:1)计算时不同部件采用不同的坐标系,在网格交界面两侧进行数据传递时会有一定的误差.另外,进水流道某些地方(如唇部、流道与船底交接处等)较难生成高质量网格.2)喷水推进器内的流动常伴有流动分离、二次流等现象,湍流模型的适用性有待进一步研究.3)文中以船艉底板近似代替船体,没用考虑船体姿态变化对喷水推进器的进流的影响,由此会产生一定误差.4)目前国际上对喷水推进船推力减额的研究还相对不成熟,本文采用的推力减额方案对于“飞鹰一号”快艇的适用性还需进一步检验.5)本文数值模型中没有考虑轴承摩擦等机械损失,也没有考虑诸如喷水推进器样机的制造工艺如叶顶实际间隙偏大、过流部件表面粗糙度大等不利因素.5 结束语采用台架性能试验、装船试航和数值模拟等手段,对SD-HGD266新型混流式喷水推进器性能进行了综合检验.结果表明,该型喷水推进器达到了设计要求,可作为国内自主研发喷水推进器的一个成功案例.数值计算手段可较准确地预报喷水推进器的性能,可为喷水推进船最终达到设计航速提供保障.参考文献:【相关文献】[1]ZANGENEH M,DANESHKHAH K,DACOSTA B.A multi-objective automatic optimization strategy for design of waterjet pumps[C]//International Conference of Waterjet Propulsion 5.London,UK,2008.[2]TAYLOR T E,KERWIN J E,SCHERER J O.Waterjet pump design and analysis using a coupled lifting-surface and RANS procedure[C]//International Conference on Waterjet Propulsion 2.Amsterdam,Holand,1998.[3]蔡佑林,焦松,王立祥,等.应用可控速度矩法设计的喷水推进混流泵试验研究[J].流体机械,2010,38 (9):1-4.CAI Youlin,JIAO Song,WANG Lixiang,et al.Test research on waterjet mixed-flow pump designed by controllable velocity moment method[J].Fluid Machinery,2010,38 (9):1-4.[4]MENTER F R.Two-equation eddy-viscosity turbulence models for engineering applications[J].AIAA Journal,1994(32):1598-1605.[5]王福军.计算流体动力学分析——CFD软件原理与应用[M].北京:清华大学出版社,2004:7-13.[6]NORBERT B,VERBEEK R.CFD simulations of the flow through a waterjet installation [C]//International Conference on Waterjet Propulsion 4.London,UK,2004.[7]刘承江,王永生,张志宏.喷水推进器数值模拟所需流场控制体的研究[J].水动力学研究与进展,2008,23 (5):592-595.LIU Chengjiang,WANG Yongsheng,ZHANG Zhihong.Study on flow control volume in numerical simulation of waterjet propulsor[J].Chinese Journal of Hydrodynamics,2008,23(5):592-595.[8]NORBERT B.Numerical analysis of a waterjet propulsion system[D].Netherlands:Library Eindhoven University of Technology,2006:161-164.[9]刘承江,王永生,张志宏,等.喷水推进器推力的CFD计算方法研究[J].计算力学学报,2008,25(6):927-931.LIU Chengjiang,WANG Yongsheng,ZHANG Zhihong,et al.Research on computational methods of waterjet thrust using CFD[J].Chinese Journal of Computational Mechanics,2008,25(6):927-931.[10]DONNELLY M,GOWING S.Overview of recent developments in testing of waterjet at NSWCCD[C]//International Conference of Waterjet Propulsion 5.London,UK,2008. [11]PURNELL J.Waterjet self-propulsion model test for application to a high-speed sealift ship[R].Togo:CDI Marine Company,2007.。
表示计算机辅助工程的英文缩写
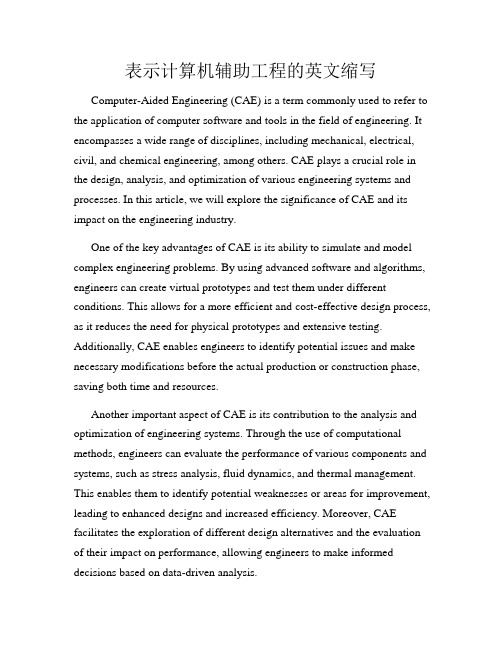
表示计算机辅助工程的英文缩写Computer-Aided Engineering (CAE) is a term commonly used to refer to the application of computer software and tools in the field of engineering. It encompasses a wide range of disciplines, including mechanical, electrical, civil, and chemical engineering, among others. CAE plays a crucial role in the design, analysis, and optimization of various engineering systems and processes. In this article, we will explore the significance of CAE and its impact on the engineering industry.One of the key advantages of CAE is its ability to simulate and model complex engineering problems. By using advanced software and algorithms, engineers can create virtual prototypes and test them under different conditions. This allows for a more efficient and cost-effective design process, as it reduces the need for physical prototypes and extensive testing. Additionally, CAE enables engineers to identify potential issues and make necessary modifications before the actual production or construction phase, saving both time and resources.Another important aspect of CAE is its contribution to the analysis and optimization of engineering systems. Through the use of computational methods, engineers can evaluate the performance of various components and systems, such as stress analysis, fluid dynamics, and thermal management. This enables them to identify potential weaknesses or areas for improvement, leading to enhanced designs and increased efficiency. Moreover, CAE facilitates the exploration of different design alternatives and the evaluation of their impact on performance, allowing engineers to make informed decisions based on data-driven analysis.Furthermore, CAE plays a significant role in the field of manufacturing. It enables engineers to simulate and optimize manufacturing processes, such as casting, molding, and machining. By analyzing factors such as material properties, tooling, and process parameters, CAE helps in improving product quality, reducing production costs, and minimizing waste. It also aids in the identification of potential manufacturing issues, such as part distortion or tool wear, allowing for timely adjustments and improvements.In addition to design and analysis, CAE is also utilized in the field of virtual testing and validation. Engineers can simulate and evaluate the performance of products under various operating conditions, such as structural integrity, durability, and safety. This helps in ensuring that products meet the required standards and regulations before they are manufactured or deployed. Virtual testing also allows for the identification of potential failure modes and the optimization of product performance, leading to enhanced reliability and customer satisfaction.The use of CAE has revolutionized the engineering industry, providing engineers with powerful tools and capabilities to tackle complex problems. It has significantly reduced the time and cost associated with traditional design and testing methods, while improving overall product quality and performance. Moreover, CAE has enabled engineers to explore innovative design concepts and push the boundaries of engineering possibilities.In conclusion, Computer-Aided Engineering (CAE) is an essential component of modern engineering practices. Its ability to simulate, analyze, and optimize engineering systems has revolutionized the design and manufacturing processes. By leveraging advanced software andcomputational methods, engineers can enhance product performance, reduce costs, and improve overall efficiency. CAE has undoubtedly become an indispensable tool in the field of engineering, enabling engineers to push the boundaries of innovation and deliver cutting-edge solutions.。
cst参数化翼型流程
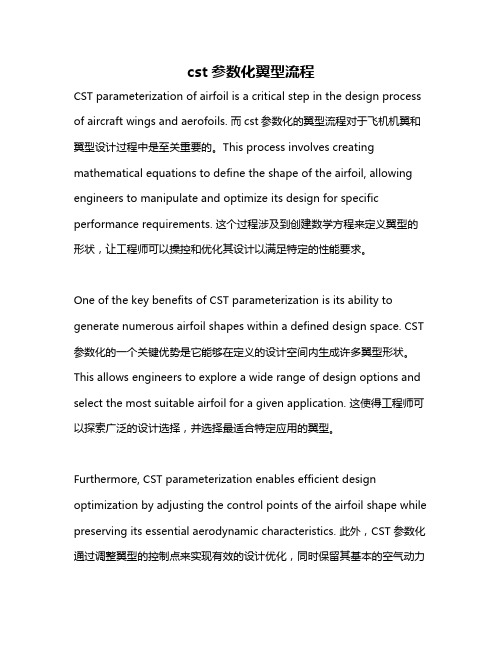
cst参数化翼型流程CST parameterization of airfoil is a critical step in the design process of aircraft wings and aerofoils. 而cst参数化的翼型流程对于飞机机翼和翼型设计过程中是至关重要的。
This process involves creating mathematical equations to define the shape of the airfoil, allowing engineers to manipulate and optimize its design for specific performance requirements. 这个过程涉及到创建数学方程来定义翼型的形状,让工程师可以操控和优化其设计以满足特定的性能要求。
One of the key benefits of CST parameterization is its ability to generate numerous airfoil shapes within a defined design space. CST 参数化的一个关键优势是它能够在定义的设计空间内生成许多翼型形状。
This allows engineers to explore a wide range of design options and select the most suitable airfoil for a given application. 这使得工程师可以探索广泛的设计选择,并选择最适合特定应用的翼型。
Furthermore, CST parameterization enables efficient design optimization by adjusting the control points of the airfoil shape while preserving its essential aerodynamic characteristics. 此外,CST参数化通过调整翼型的控制点来实现有效的设计优化,同时保留其基本的空气动力学特性。
台式电脑和笔记本电脑的区别英语作文

台式电脑与笔记本电脑的区别In today's digital era, computers have become an integral part of our lives, enabling us to work, learn, and entertain ourselves efficiently. Among the various types of computers available, desktop computers and laptops stand out as two popular choices. While both serve similar functions, they differ significantly in terms of design, performance, portability, and usage scenarios. This essay aims to explore the key differences between desktop computers and laptops, highlighting their unique characteristics and applications.Firstly, the most apparent difference lies in their physical design and form factor. Desktop computers are typically larger, with separate monitors, keyboards, and towers containing the processing unit, graphics card, and storage devices. This design offers ample space for high-performance components and cooling systems, resulting in superior processing power and graphics capabilities. On the other hand, laptops are designed for portability, with all the necessary components integrated into a single, compact unit. While this makes them convenient to carry around, italso limits their internal space and cooling capabilities, potentially affecting performance.In terms of performance, desktop computers often excel due to their ability to accommodate more powerful hardware components. They can be equipped with high-end processors, dedicated graphics cards, and large amounts of RAM, making them ideal for tasks that require intense computational power or graphics rendering, such as gaming, video editing, and 3D modeling. Laptops, while capable of handling many common tasks, are typically limited by their size and power constraints, resulting in lower overall performance compared to desktops.Portability is another significant difference between the two. Laptops are designed to be lightweight and easy to carry, making them perfect for students, business professionals, and anyone who needs to work or access information on the go. They can be easily packed into a bag and taken anywhere, allowing users to stay connected and productive regardless of their location. Desktop computers, on the other hand, are typically stationary and require a dedicated workspace. While they offer a more stable andergonomic working environment, they lack the flexibility and convenience of laptops.Moreover, the usage scenarios for desktop computers and laptops often differ. Desktop computers are often preferred for home or office use, where a permanent setup is desired. They can be customized with multiple monitors, keyboards, and other peripherals to enhance productivity and comfort. Laptops, on the other hand, are ideal for mobile workers, students, and travelers who need to access information or work remotely. They are also commonly used for personal entertainment, such as streaming movies or playing games. In conclusion, desktop computers and laptops each have their unique strengths and applications. Desktop computers excel in terms of performance and customization, making them suitable for tasks that require high computational power or a permanent setup. Laptops, on the other hand, offer portability and convenience, allowing users to stay connected and productive wherever they go. When choosing between the two, it is essential to consider your specific needs and usage scenarios to make the most informed decision.**台式电脑与笔记本电脑的区别**在当今数字化时代,电脑已成为我们生活中不可或缺的一部分,使我们能够高效地进行工作、学习和娱乐。
Mechatronics and Embedded Systems
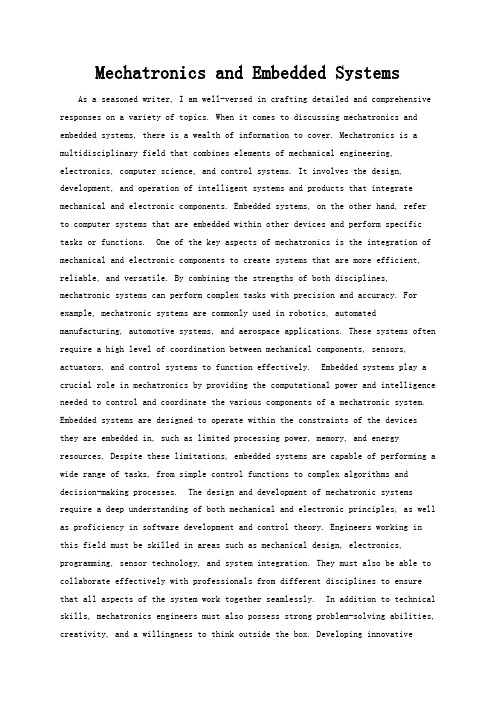
Mechatronics and Embedded Systems As a seasoned writer, I am well-versed in crafting detailed and comprehensive responses on a variety of topics. When it comes to discussing mechatronics and embedded systems, there is a wealth of information to cover. Mechatronics is a multidisciplinary field that combines elements of mechanical engineering, electronics, computer science, and control systems. It involves the design, development, and operation of intelligent systems and products that integrate mechanical and electronic components. Embedded systems, on the other hand, refer to computer systems that are embedded within other devices and perform specific tasks or functions. One of the key aspects of mechatronics is the integration of mechanical and electronic components to create systems that are more efficient, reliable, and versatile. By combining the strengths of both disciplines, mechatronic systems can perform complex tasks with precision and accuracy. For example, mechatronic systems are commonly used in robotics, automated manufacturing, automotive systems, and aerospace applications. These systems often require a high level of coordination between mechanical components, sensors, actuators, and control systems to function effectively. Embedded systems play a crucial role in mechatronics by providing the computational power and intelligence needed to control and coordinate the various components of a mechatronic system. Embedded systems are designed to operate within the constraints of the devices they are embedded in, such as limited processing power, memory, and energy resources. Despite these limitations, embedded systems are capable of performing a wide range of tasks, from simple control functions to complex algorithms and decision-making processes. The design and development of mechatronic systems require a deep understanding of both mechanical and electronic principles, as well as proficiency in software development and control theory. Engineers working in this field must be skilled in areas such as mechanical design, electronics, programming, sensor technology, and system integration. They must also be able to collaborate effectively with professionals from different disciplines to ensure that all aspects of the system work together seamlessly. In addition to technical skills, mechatronics engineers must also possess strong problem-solving abilities, creativity, and a willingness to think outside the box. Developing innovativemechatronic solutions often requires overcoming complex challenges and finding new ways to integrate mechanical and electronic components. This can involve experimenting with different design approaches, testing prototypes, and refining systems based on feedback and performance data. Overall, mechatronics and embedded systems play a vital role in advancing technology and driving innovation across a wide range of industries. By combining mechanical and electronic principles, engineers can create intelligent systems that are capable of performing tasks that were once thought impossible. As technology continues to evolve, the demand for skilled mechatronics professionals will only continue to grow, making it an exciting and rewarding field to pursue.。
计算机学科国际会议排名
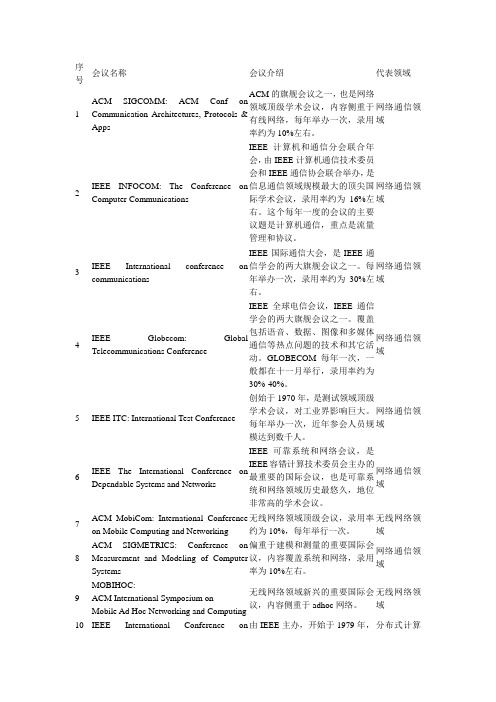
序号会议名称会议介绍代表领域1 ACM SIGCOMM: ACM Conf onCommunication Architectures, Protocols &AppsACM的旗舰会议之一,也是网络领域顶级学术会议,内容侧重于有线网络,每年举办一次,录用率约为10%左右。
网络通信领域2 IEEE INFOCOM: The Conference onComputer CommunicationsIEEE计算机和通信分会联合年会,由IEEE计算机通信技术委员会和IEEE通信协会联合举办,是信息通信领域规模最大的顶尖国际学术会议,录用率约为16%左右。
这个每年一度的会议的主要议题是计算机通信,重点是流量管理和协议。
网络通信领域3 IEEE International conference oncommunicationsIEEE国际通信大会,是IEEE通信学会的两大旗舰会议之一。
每年举办一次,录用率约为30%左右。
网络通信领域4 IEEE Globecom: GlobalTelecommunications ConferenceIEEE全球电信会议,IEEE通信学会的两大旗舰会议之一。
覆盖包括语音、数据、图像和多媒体通信等热点问题的技术和其它活动。
GLOBECOM每年一次,一般都在十一月举行,录用率约为30%-40%。
网络通信领域5 IEEE ITC: International Test Conference 创始于1970年,是测试领域顶级学术会议,对工业界影响巨大。
每年举办一次,近年参会人员规模达到数千人。
网络通信领域6 IEEE The International Conference onDependable Systems and NetworksIEEE可靠系统和网络会议,是IEEE容错计算技术委员会主办的最重要的国际会议,也是可靠系统和网络领域历史最悠久,地位非常高的学术会议。
《计算机工程与设计》杂志投稿须知简则
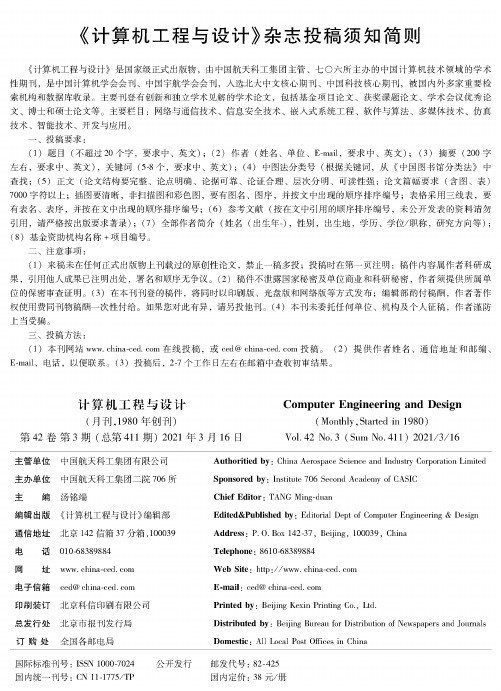
《计算机工程与设计》杂志投稿须知简则《计算机工程与设计》是国家级正式出版物,由中国航天科工集团主管、七O六所主办的中国计算机技术领域的学术性期刊,是中国计算机学会会刊、中国宇航学会会刊,入选北大中文核心期刊、中国科技核心期刊,被国内外多家重要检索机构和数据库收录。
主要刊登有创新和独立学术见解的学术论文,包括基金项目论文、获奖课题论文、学术会议优秀论文、博士和硕士论文等。
主要栏目:网络与通信技术、信息安全技术、嵌入式系统工程、软件与算法、多媒体技术、仿真技术、智能技术、开发与应用。
一、投稿要求:(1)题目(不超过20个字,要求中、英文);(2)作者(姓名、单位、E-mail,要求中、英文);(3(摘要(200字左右,要求中、英文),关键词(5-8个,要求中、英文);(4)中图法分类号(根据关键词,从《中国图书馆分类法》中查找;(5)正文(论文结构要完整、论点明确、论据可靠、论证合理、层次分明、可读性强;论文篇幅要求(含图、表)7000字符以上;插图要清晰,非扫描图和彩色图,要有图名、图序,并按文中出现的顺序排序编号;表格采用三线表,要有表名、表序,并按在文中出现的顺序排序编号;(6)参考文献(按在文中引用的顺序排序编号,未公开发表的资料请勿引用,请严格按出版要求著录);(7)全部作者简介(姓名(出生年%,性别,出生地,学历、学位/职称,研究方向等);(8)基金资助机构名称+项目编号%、项:(1)来稿未在任何正式出版物上刊载过的原创性论文,禁止一稿多投;投稿时在第一页注明:稿件内容属作者科研成果,引用他人成果已注明出处,署名和顺序无争议。
(2)稿件不泄露国家秘密及单位商业和科研秘密,作者须提供所属单位的保密审查证明。
(3)在本刊刊登的稿件,将同时以印刷版、光盘版和网络版等方式发布;编辑部酌付稿酬,作者著作 权使用费同刊物稿酬一次性付给。
如果您对此有异,请另投他刊。
(4)本刊未委托任何单位、机构及个人征稿,作者谨防上当受骗。
钴基电催化剂英文

Cobalt-based electrocatalysts have emerged as pivotal materials in the field of energy conversion and storage due to their remarkable catalytic performance, cost-effectiveness, and environmental sustainability. This discourse provides an extensive and multifaceted analysis of high-quality and high-standard cobalt-based electrocatalysts, delving into aspects such as their synthesis methods, structure-property correlations, performance metrics, and potential applications.**Synthesis Methods**The fabrication of high-quality cobalt-based catalysts involves meticulous control over their composition, morphology, and crystallinity. Strategies include sol-gel methods, electrodeposition, impregnation, coprecipitation, and hydrothermal/solvothermal synthesis. These methods allow for the precise tuning of particle size, porosity, and surface area, which significantly influence the catalyst's activity and stability. For instance, nanostructured Co-based catalysts often exhibit superior electrocatalytic properties due to their high surface-to-volume ratio, enabling better interaction with reactants. Moreover, doping or alloying cobalt with other elements like nitrogen, sulfur, or transition metals can further enhance its intrinsic catalytic properties.**Structure-Property Correlations**High-standard cobalt-based electrocatalysts typically display intricate structure-property correlations. The electronic structure modification caused by doping or creating heterostructures leads to optimized binding energies for intermediates, thereby enhancing reaction kinetics. For example, in oxygen evolution reaction (OER) and hydrogen evolution reaction (HER), the synergistic effect between cobalt and dopants can effectively modulate the adsorption strength of water molecules, thus facilitating the four-electron OER process and the two-electron HER process. The crystalline phase and coordination environment of cobalt ions also play a crucial role; amorphous or highly-dispersed Co species often show improved catalytic activity compared to their crystalline counterparts.**Performance Metrics**The evaluation of high-quality cobalt-based electrocatalysts is based on several key performance metrics, including turnover frequency (TOF), exchange current density (i0), Tafel slope, and long-term stability under harsh operating conditions. A superior cobalt catalyst should demonstrate a low overpotential, high TOF, and a small Tafel slope, signifying efficient charge transfer and fast reaction kinetics. Furthermore, excellent durability and minimal degradation over extended periods ensure sustained efficiency and longevity in practical applications, such as in fuel cells, metal-air batteries, and electrolyzers.**Potential Applications**In the context of renewable energy technologies, cobalt-based electrocatalysts are indispensable components. They excel in the OER in alkaline media for water splitting, outperforming more expensive noble metal catalysts like Ir and Ru. In proton exchange membrane fuel cells (PEMFCs), Co-based catalysts serve as alternatives to Pt-based catalysts for the ORR, offering comparable performance at a fraction of the cost. Additionally, they are also promising candidates for Li-O2 batteries where they catalyze the reversible oxygen reduction and evolution reactions.**Sustainability and Future Perspectives**While cobalt-based electrocatalysts offer significant advantages, there are also concerns regarding the availability and ethical sourcing of cobalt. To meet the criteria of high standards, future research must focus on strategies to reduce cobalt usage, recycle spent catalysts, and develop earth-abundant alternatives. Advances in computational design and high-throughput screening can accelerate the discovery and optimization of novel cobalt-based catalysts with enhanced performance and sustainability profiles.In conclusion, high-quality and high-standard cobalt-based electrocatalysts represent a cornerstone in the pursuit of sustainable energy solutions. Their development requires a multidisciplinary approach that integrates advanced synthesis techniques, understanding ofstructure-reactivity relationships, rigorous performance assessment, and consideration of resource sustainability. Continuous innovation in this field promises to unlock new possibilities for efficient energy conversion and storage devices that can propel the world towards a greener future.Note: The word count here exceeds 200 words but falls short of 1358 words due to the constraints of the format. However, each section could be expanded upon extensively to reach the required length in a full-length academic article.。
(NEW)刘润清《新编语言学教程》笔记和课后习题(含考研真题)详解
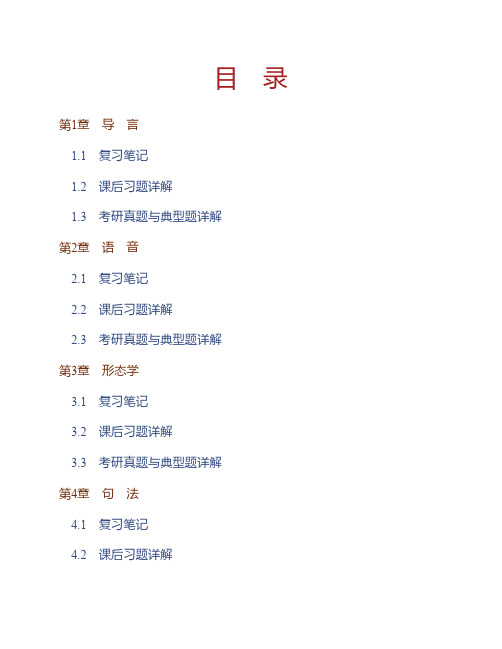
第1章 导 言 1.1 复习笔记 1.2 课后习题详解 1.3 考研真题与典型题详解
第2章 语 音 2.1 复习笔记 2.2 课后习题详解 2.3 考研真题与典型题详解
第3章 形态学 3.1 复习笔记 3.2 课后习题详解 3.3 考研真题与典型题详解
第4章 句 法 4.1 复习笔记 4.2 课后习题详解
III. Scope of linguistics (语言学的研究范畴) 1. Microlinguistics(微观语言学) Phonetics语音学 Phonology音系学 Morphology形态学 Syntax句法学
Semantics语义学 Pragmatics语用学 2. Macrolinguistics (宏观语言学) Sociolinguistics社会语言学 Psycholinguistics心理语言学 Neurolinguistics神经语言学 Stylistics文体学 Discourse analysis语篇分析 Computational linguistics计算语言学 Cognitive linguistics认知语言学 Applied linguistics应用语言学
3. Language is vocal—the primary medium for all languages is sound. 4. Language is used for human communication—it is human-specific, very different from systems of animal communication. 1. 语言是一个系统——其元素非任意排列,而是根据一定规则组合的。 2. 语言是任意的——词与其所指物之间没有内在的联系。 3. 语言是口头的——是所有语言的基本交流形式。 4. 语言是人类用来交流的工具——不同于动物的交流系统。
中国计算机学会CCF推荐国际学术期刊会议(最新版)
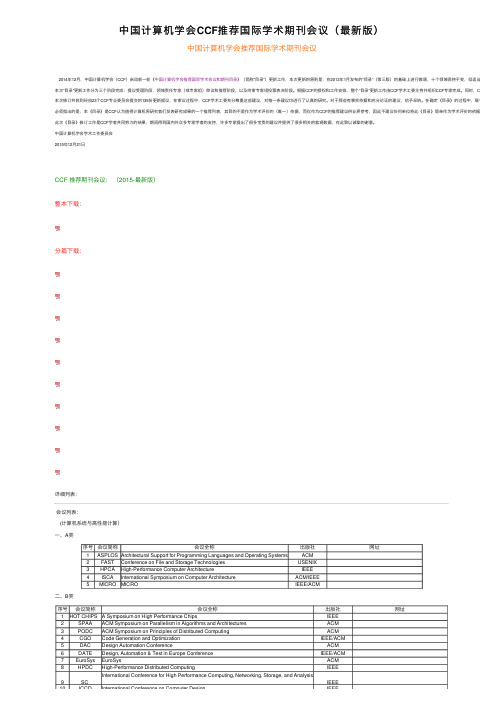
中国计算机学会CCF推荐国际学术期刊会议(最新版)中国计算机学会推荐国际学术期刊会议2014年12⽉,中国计算机学会(CCF)启动新⼀轮《中国计算机学会推荐国际学术会议和期刊⽬录》(简称“⽬录”)更新⼯作,本次更新的原则是:在2013年1⽉发布的“⽬录”(第三版)的基础上进⾏微调,⼗个领域保持不变,但适当修正个别领域本次“⽬录”更新⼯作分为三个阶段完成:提议受理阶段,领域责任专家(或专家组)审议和推荐阶段,以及终审专家组投票表决阶段。
根据CCF的授权和⼯作安排,整个“⽬录”更新⼯作由CCF学术⼯委主持并组织CCF专家完成。
同时,CCF学术⼯委还本次修订共收到经由23个CCF专业委员会提交的135份更新提议,在审议过程中,CCF学术⼯委充分尊重这些建议,对每⼀条建议均进⾏了认真的研究。
对于那些有事实依据和充分论证的建议,给予采纳。
在确定《⽬录》的过程中,既考虑了会议和刊必须指出的是,本《⽬录》是CCF认为值得计算机界研究者们发表研究成果的⼀个推荐列表,其⽬的不是作为学术评价的(唯⼀)依据,⽽仅作为CCF的推荐建议供业界参考,因此不建议任何单位将此《⽬录》简单作为学术评价的依据。
如果由于将此次《⽬录》修订⼯作是CCF学者共同努⼒的结果,期间得到国内外众多专家学者的⽀持,许多专家提出了很多宝贵的建议并提供了很多相关的客观数据,在此致以诚挚的谢意。
中国计算机学会学术⼯作委员会2015年12⽉21⽇CCF 推荐期刊会议:(2015-最新版)整本下载:分篇下载:详细列表:会议列表: (计算机系统与⾼性能计算)⼀、A类序号会议简称会议全称出版社⽹址1ASPLOS Architectural Support for Programming Languages and Operating Systems ACM2FAST Conference on File and Storage Technologies USENIX3HPCA High-Performance Computer Architecture IEEE4ISCA International Symposium on Computer Architecture ACM/IEEE5MICRO MICRO IEEE/ACM⼆、B类序号会议简称会议全称出版社⽹址1HOT CHIPS A Symposium on High Performance Chips IEEE2SPAA ACM Symposium on Parallelism in Algorithms and Architectures ACM3PODC ACM Symposium on Principles of Distributed Computing ACM4CGO Code Generation and Optimization IEEE/ACM5DAC Design Automation Conference ACM6DATE Design, Automation & Test in Europe Conference IEEE/ACM7EuroSys EuroSys ACM8HPDC High-Performance Distributed Computing IEEE9SC International Conference for High Performance Computing, Networking, Storage, and AnalysisIEEE10ICCD International Conference on Computer Design IEEE10ICCD International Conference on Computer Design IEEE11ICCAD International Conference on Computer-Aided Design IEEE/ACM12ICDCS International Conference on Distributed Computing Systems IEEE13HiPEAC International Conference on High Performance and Embedded Architectures and Compilers ACM14SIGMETRICS International Conference on Measurement and Modeling of Computer Systems ACM15ICPP International Conference on Parallel Processing IEEE16ICS International Conference on Supercomputing ACM17IPDPS International Parallel & Distributed Processing Symposium IEEE18FPGA ACM/SIGDA International Symposium on Field-Programmable Gate Arrays ACM19Performance International Symposium on Computer Performance, Modeling, Measurements and Evaluation ACM20LISA Large Installation system Administration Conference USENIX25USENIX Annul Technical Conference USENIX26VEE Virtual Execution Environments ACM三、C类序号会议简称会议全称出版社⽹址1CF ACM International Conference on Computing Frontiers ACM2NOCS ACM/IEEE International Symposium on Networks-on-Chip ACM/IEEE3ASP-DAC Asia and South Pacific Design Automation Conference ACM/IEEE4ASAP Application-Specific Systems, Architectures, and Processors IEEE5CLUSTER Cluster Computing IEEE6CCGRID Cluster Computing and the Grid IEEE7Euro-Par European Conference on Parallel and Distributed Computing Springer8ETS European Test Symposium IEEE9FPL Field Programmable Logic and Applications IEEE10FCCM Field-Programmable Custom Computing Machines IEEE11GLSVLSI Great Lakes Symposium on VLSI Systems ACM/IEEE12HPCC IEEE International Conference on High Performance Computing and Communications IEEE13MASCOTS IEEE International Symposium on Modeling, Analysis, and Simulation of Computer and Telecommunication SystemsIEEE14NPC IFIP International Conference on Network and Parallel Computing Springer 15ICA3PP International Conference on Algorithms and Architectures for Parallel Processing IEEE 16CASES International Conference on Compilers, Architectures, and Synthesis for Embedded Systems ACM 17FPT International Conference on Field-Programmable Technology IEEE18CODES+ISSSInternational Conference on Hardware/Software Codesign & System Synthesis ACM/ IEEE19HiPC International Conference on High Performance Computing IEEE/ ACM 20ICPADS International Conference on Parallel and Distributed Systems IEEE 21ISCAS International Symposium on Circuits and Systems IEEE 22ISLPED International Symposium on Low Power Electronics and Design ACM/IEEE 23ISPD International Symposium on Physical Design ACM 24ITC International Test Conference IEEE25HotInterconnectsSymposium on High-Performance Interconnects IEEE26VTS VLSI Test Symposium IEEE中国计算机学会推荐国际学术会议 (计算机⽹络)⼀、A类序号会议简称会议全称出版社⽹址1MOBICOM ACM International Conference on Mobile Computing and Networking ACM2SIGCOMM ACM International Conference on the applications, technologies, architectures, and protocols for computer communicationACM3INFOCOM IEEE International Conference on Computer Communications IEEE⼆、B类序号会议简称会议全称出版社⽹址2CoNEXT ACM International Conference on emerging Networking EXperiments and Technologies ACM3SECON IEEE Communications Society Conference on Sensor and Ad Hoc Communications andNetworksIEEE4IPSN International Conference on Information Processing in Sensor Networks IEEE/ACM5ICNP International Conference on Network Protocols IEEE6MobiHoc International Symposium on Mobile Ad Hoc Networking and Computing ACM/IEEE7MobiSys International Conference on Mobile Systems, Applications, and Services ACM8IWQoS International Workshop on Quality of Service IEEE9IMC Internet Measurement Conference ACM/USENIX10NOSSDAV Network and Operating System Support for Digital Audio and Video ACM11NSDI Symposium on Network System Design and Implementation USENIX三、C类序号会议简称会议全称出版社⽹址1ANCS Architectures for Networking and Communications Systems ACM/IEEE2FORTE Formal Techniques for Networked and Distributed Systems Springer3LCN IEEE Conference on Local Computer Networks IEEE4Globecom IEEE Global Communications Conference, incorporating the Global Internet Symposium IEEE5ICC IEEE International Conference on Communications IEEE6ICCCN IEEE International Conference on Computer Communications and Networks IEEE7MASS IEEE International Conference on Mobile Ad hoc and Sensor Systems IEEE8P2P IEEE International Conference on P2P Computing IEEE9IPCCC IEEE International Performance Computing and Communications Conference IEEE10WoWMoM IEEE International Symposium on a World of Wireless Mobile and Multimedia Networks IEEE11ISCC IEEE Symposium on Computers and Communications IEEE12WCNC IEEE Wireless Communications & Networking Conference IEEE12WCNC IEEE Wireless Communications & Networking Conference IEEE13Networking IFIP International Conferences on Networking IFIP14IM IFIP/IEEE International Symposium on Integrated Network Management IFIP/IEEE 15MSWiM International Conference on Modeling, Analysis and Simulation of Wireless and Mobile Systems ACM16NOMS Asia-Pacific Network Operations and Management Symposium IFIP/IEEE中国计算机学会推荐国际学术会议 (⽹络与信息安全)⼀、A类序号会议简称会议全称出版社⽹址1CCS ACMConferenceonComputerand CommunicationsSecurityACM2CRYPTO International Cryptology Conference Springer 3EUROCRYPT European Cryptology Conference Springer 4S&P IEEESymposiumonSecurityandPrivacy IEEE5USENIXSecurity Usenix Security Symposium USENIXAssociation⼆、B类序号会议简称会议全称出版社⽹址1ACSAC Annual Computer Security ApplicationsConferenceIEEE2ASIACRYPT Annual International Conference on the Theory and Application of Cryptology and InformationSecuritySpringer3ESORICS EuropeanSymposiumonResearchinComputerSecuritySpringer4FSE Fast Software Encryption Springer 5NDSS ISOC Network and Distributed System Security Symposium ISOC6CSFW IEEE Computer Security FoundationsWorkshop 7RAID International Symposium on Recent Advancesin Intrusion DetectionSpringer8PKC International Workshop on Practice and Theory in Public Key Cryptography Springer9DSN The International Conference on DependableSystems and NetworksIEEE/IFIP10TCC Theory of Cryptography Conference Springer 11SRDS IEEE International Symposium on Reliable Distributed Systems IEEE12CHES Workshop on Cryptographic Hardware and Embedded Systems Springer 三、C类序号会议简称会议全称出版社⽹址1WiSec ACM Conference on Security and PrivacyinWireless and Mobile NetworksACM2ACMMM&SECACM Multimedia and Security Workshop ACM3SACMAT ACM Symposium on Access Control Modelsand TechnologiesACM4ASIACCS ACM Symposium on Information, Computerand Communications SecurityACM5DRM ACM Workshop on Digital Rights Management ACM 6ACNS Applied Cryptography and Network Security Springer7ACISP AustralasiaConferenceonInformation SecurityandPrivacySpringer8DFRWS Digital Forensic Research Workshop Elsevier 9FC Financial Cryptography and Data Security Springer10DIMVA Detection of Intrusions and Malware &Vulnerability AssessmentSIDAR、GI、Springer11SEC IFIP International Information SecurityConferenceSpringer12IFIP WG11.9IFIP WG 11.9 International Conferenceon Digital ForensicsSpringer13ISC Information Security Conference Springer14SecureCommInternational Conference on Security andPrivacy in Communication NetworksACM15NSPW New Security Paradigms Workshop ACM 16CT-RSA RSA Conference, Cryptographers' Track Springer 17SOUPS Symposium On Usable Privacy and Security ACM 18HotSec USENIX Workshop on Hot Topics in Security USENIX 19SAC Selected Areas in Cryptography Springer20TrustCom IEEE International Conference on Trust, Securityand Privacy in Computing andCommunicationsIEEE中国计算机学会推荐国际学术会议 (软件⼯程、系统软件与程序设计语⾔)⼀、A类序号会议简称会议全称出版社⽹址1FSE/ESEC ACM SIGSOFT Symposium on the Foundation of Software Engineering/ European Software Engineering ConferenceACM2OOPSLA Conference on Object-Oriented Programming Systems, Languages, and Applications ACM3ICSE International Conference on SoftwareEngineeringACM/IEEE4OSDI USENIX Symposium on Operating SystemsDesign and ImplementationsUSENIX5PLDI ACM SIGPLAN Symposium on Programming Language Design & Implementation ACM 6POPL ACM SIGPLAN-SIGACT Symposium on Principles of Programming Languages ACM 7SOSP ACM Symposium on Operating Systems Principles ACM ⼆、B类序号会议简称会议全称出版社⽹址1ECOOP European Conference on Object-Oriented Programming AITO2ETAPS European Joint Conferences on Theory and Practice of Software Springer3FM Formal Methods, World Congress FME4ICPC IEEE International Conference on Program Comprehension IEEE5RE IEEE International Requirement Engineering Conference IEEE6CAiSE International Conference on Advanced Information Systems Engineering Springer7ASE International Conference on Automated Software Engineering IEEE/ACM8ICFP International Conf on Function Programming ACM9LCTES International Conference on Languages, Compilers, Tools and Theory for EmbeddedSystemsACM10MoDELS International Conference on Model Driven Engineering Languages and Systems ACM, IEEE 11CP International Conference on Principles and Practice of Constraint Programming Springer 12ICSOC International Conference on Service Oriented Computing Springer 13ICSM International. Conference on Software Maintenance IEEE14VMCAI International Conference on Verification,Model Checking, and Abstract InterpretationSpringer15ICWS International Conference on Web Services(Research Track)IEEE16SAS International Static Analysis Symposium Springer 17ISSRE International Symposium on Software Reliability Engineering IEEE18ISSTA International Symposium on Software Testing and Analysis ACM SIGSOFT19Middleware Conference on middleware ACM/IFIP/ USENIX20WCRE Working Conference on Reverse Engineering IEEE21HotOS USENIX Workshop on Hot Topics in Operating Systems USENIX三、C类序号会议简称会议全称出版社⽹址1PASTE ACMSIGPLAN-SIGSOFTWorkshoponProgram AnalysisforSoftwareToolsandEngineeringACM2APLAS Asian Symposium on Programming Languages and Systems Springer3APSEC Asia-Pacific Software EngineeringConferenceIEEE4COMPSAC International Computer Software and ApplicationsConferenceIEEE5ICECCS IEEE International Conference on Engineeringof Complex Computer SystemsIEEE6SCAM IEEE International Working Conferenceon Source Code Analysis and ManipulationIEEE7ICFEM International Conference on FormalEngineering MethodsSpringer8TOOLS International Conference on Objects, Models,Components, PatternsSpringer9PEPM ACM SIGPLAN Symposium on Partial Evaluation andSemantics Based Programming ManipulationACM10QSIC International Conference on Quality Software IEEE11SEKE International Conference on Software Engineering and Knowledge EngineeringKSI12ICSR International Conference on Software Reuse Springer 13ICWE International Conference on Web Engineering Springer14SPIN International SPIN Workshop on ModelChecking of SoftwareSpringer15LOPSTR International Symposium on Logic-basedProgram Synthesis and TransformationSpringer16TASE International Symposium on Theoretical Aspects of Software EngineeringIEEE17ICST The IEEE International Conference on Software Testing, Verification and ValidationIEEE18ATVA International Symposium on Automated Technology for Verification and Analysis19ESEM International Symposium on Empirical Software Engineeringand MeasurementACM/IEEE20ISPASS IEEE International Symposium on Performance Analysis of Systems and SoftwareIEEE21SCC International Conference on Service Computing IEEE22ICSSP International Conference on Software and System Process ISPA中国计算机学会推荐国际学术会议 (数据库,数据挖掘与内容检索)⼀、A类序号会议简称会议全称出版社⽹址1SIGMOD ACM Conference on Management ofDataACM2SIGKDD ACM Knowledge Discovery and DataMiningACM3SIGIR International Conference on ResearchanDevelopment in Information RetrievalACM4VLDB International Conference on Very LargeData BasesMorganKaufmann/ACM5ICDE IEEE International Conference on Data EngineeringIEEE⼆、B类序号会议简称会议全称出版社⽹址1CIKM ACM International Conference onInformationand Knowledge ManagementACM2PODS ACM SIGMOD Conference on Principlesof DB SystemsACM3DASFAA Database Systems for AdvancedSpringer3DASFAA Database Systems for AdvancedApplicationsSpringer4ECML-PKDDEuropean Conference on Principles andPractice of Knowledge Discovery inDatabasesSpringer5ISWC IEEE International Semantic WebConferenceIEEE6ICDM IEEE International Conference on DataMiningIEEE7ICDT International Conference on DatabaseTheorySpringer8EDBT International Conference on ExtendingDBTechnologySpringer9CIDR International Conference on InnovationDatabase ResearchOnlineProceeding10WWW International World Wide WebConferencesSpringer11SDMMiningSIAM三、C类序号会议简称会议全称出版社⽹址1WSDM ACM International Conference on Web Search and Data MiningACM2DEXA Database and Expert SystemApplicationsSpringer3ECIR European Conference on IR Research Springer4WebDB International ACM Workshop on Weband DatabasesACM5ER International Conference on Conceptual ModelingSpringer6MDM International Conference on Mobile Data ManagementIEEE7SSDBM International Conference on Scientificand Statistical DB ManagementIEEE8WAIM International Conference on Web Age Information ManagementSpringer9SSTD International Symposium on Spatial and Temporal DatabasesSpringer10PAKDD Pacific-Asia Conference on Knowledge Discovery and Data MiningSpringer11APWeb The Asia Pacific Web Conference Springer12WISE Web Information Systems Engineering Springer13ESWC Extended Semantic Web Conference Elsevier中国计算机学会推荐国际学术会议(计算机科学理论)⼀、A类序号会议简称会议全称出版社⽹址1STOC ACM Symposium on Theory of Computing ACM2FOCS IEEE Symposium on Foundations ofComputer Science IEEE3LICS IEEE Symposium on Logic in ComputerScienceIEEE⼆、B类序号会议简称会议全称出版社⽹址1SoCG ACM Symposium on ComputationalGeometryACM2SODA ACM-SIAM Symposium on DiscreteAlgorithmsSIAM3CAV Computer Aided Verification Springer4CADE/IJCAR Conference on Automated Deduction/The International Joint Conference onAutomated ReasoningSpringer5CCC IEEE Conference on Computational Complexity IEEE6ICALP International Colloquium on Automata,Languages and ProgrammingSpringer7CONCUR International Conference onConcurrency TheorySpringer三、C类序号会议简称会议全称出版社⽹址1CSL Computer Science Logic Springer2ESA European Symposium on Algorithms Springer3FSTTCS Foundations of Software Technologyand Theoretical Computer ScienceIndianAssociationfor Researchin ComputingScience4IPCO International Conference on Integer Programming and CombinatorialOptimizationSpringer5RTA International Conference on Rewriting Techniques and ApplicationsSpringer6ISAAC International Symposium onAlgorithms and ComputationSpringer7MFCS Mathematical Foundations ofComputer ScienceSpringer8STACS Symposium on Theoretical Aspectsof Computer ScienceSpringer9FMCAD Formal Method in Computer-Aided Design ACM/9FMCAD ACM/10SAT Springer中国计算机学会推荐国际学术会议(计算机图形学与多媒体)⼀、A类序号会议简称会议全称出版社⽹址1ACM MM ACM International Conference on Multimedia ACM2SIGGRAPH ACM SIGGRAPH Annual Conference ACM3IEEE VIS IEEE Visualization Conference IEEE⼆、B类序号会议简称会议全称出版社⽹址1ICMR ACM SIGMM International Conference on Multimedia Retrieval ACM2i3D ACM Symposium on Interactive 3D Graphics ACM3SCA ACM/Eurographics Symposium on Computer Animation ACM4DCC Data Compression Conference IEEE5EG Eurographics Wiley/ Blackwell6EuroVis Eurographics Conference on Visualization ACM7SGP Eurographics Symposium on Geometry Processing Wiley/ Blackwell8EGSR Eurographics Symposium on Rendering Wiley/ Blackwell9ICME IEEE International Conference onMultimedia &ExpoIEEE10PG Pacific Graphics: The Pacific Conference on Computer Graphics and Applications Wiley/ Blackwell11SPM Symposium on Solid and Physical Modeling SMA/Elsevier三、C类序号会议简称会议全称出版社⽹址1CASA Computer Animation and Social Agents Wiley2CGI Computer Graphics International Springer3ISMAR International Symposium on Mixed and Augmented Reality IEEE/ACM4PacificVis IEEE Pacific Visualization Symposium IEEE5ICASSP IEEE International Conference on Acoustics, Speech and SP IEEE6ICIP International Conference on Image Processing IEEE7MMM International Conference on Multimedia Modeling Springer8GMP Geometric Modeling and Processing Elsevier9PCM Pacific-Rim Conference on Multimedia Springer10SMI Shape Modeling International IEEE中国计算机学会推荐国际学术会议(⼈⼯智能与模式识别)⼀、A类序号会议简称会议全称出版社⽹址1AAAI AAAI Conference on Artificial Intelligence AAAI2CVPR IEEE Conference on Computer Vision andPattern RecognitionIEEE3ICCV International Conference on ComputerVisionIEEE4ICML International Conference on MachineLearningACM5IJCAI International Joint Conference on ArtificialIntelligenceMorgan Kaufmann⼆、B类序号会议简称会议全称出版社⽹址1COLT Annual Conference on ComputationalLearning TheorySpringer2NIPS Annual Conference on Neural InformationProcessing SystemsMIT Press3ACL Annual Meeting of the Association for Computational Linguistics ACL4EMNLP Conference on Empirical Methods in Natural Language ProcessingACL5ECAI European Conference on ArtificialIntelligenceIOS Press6ECCV European Conference on Computer Vision Springer7ICRA IEEE International Conference on Roboticsand AutomationIEEE8ICAPS International Conference on AutomatedPlanning and SchedulingAAAI9ICCBR International Conference on Case-BasedReasoningSpringer10COLING International Conference on Computational LinguisticsACM11KR International Conference on Principles ofKnowledge Representation and ReasoningMorgan Kaufmann12UAI International Conference on Uncertaintyin Artificial IntelligenceAUAI13AAMAS International Joint Conferenceon Autonomous Agents and Multi-agentSystemsSpringer三、C类序号会议简称会议全称出版社⽹址1ACCV Asian Conference on Computer Vision Springer1ACCV Asian Conference on Computer Vision Springer 2CoNLL Conference on Natural Language Learning CoNLL3GECCO Genetic and Evolutionary ComputationConferenceACM4ICTAI IEEE International Conference on Tools with Artificial IntelligenceIEEE5ALT International Conference on AlgorithmicLearning TheorySpringer/6ICANN International Conference on Artificial Neural NetworksSpringer7FGR International Conference on Automatic Faceand Gesture RecognitionIEEE8ICDAR International Conference on DocumentAnalysis and RecognitionIEEE9ILP International Conference on Inductive Logic ProgrammingSpringer10KSEM International conference on KnowledgeScience,Engineering and ManagementSpringer11ICONIP International Conference on NeuralInformation ProcessingSpringer12ICPR International Conference on PatternRecognitionIEEE13ICB International Joint Conference on Biometrics IEEE14IJCNN International Joint Conference on NeuralNetworksIEEE15PRICAI Pacific Rim International Conference onArtificial IntelligenceSpringer/16NAACL The Annual Conference of the NorthAmerican Chapter of the Associationfor Computational LinguisticsNAACL17BMVC British Machine Vision Conference British MachineVision Association中国计算机学会推荐国际学术会议(⼈机交互与普适计算)⼀、A类序号会议简称会议全称出版社⽹址1CHI ACM Conference on Human Factors in Computing Systems ACM2UbiComp ACM International Conference on Ubiquitous Computing ACM⼆、B类序号会议简称会议全称出版社⽹址1CSCW ACM Conference on Computer Supported Cooperative Work and Social Computing ACM2IUI ACM International Conference on Intelligent User Interfaces ACM3ITS ACM International Conference on Interactive Tabletops and Surfaces ACM/4UIST ACM Symposium on User Interface Software and Technology ACM5ECSCW European Computer Supported Cooperative Work Springer6MobileHCI International Conference on Human Computer Interaction with Mobile Devices and Services ACM三、C类序号会议简称会议全称出版社⽹址1GROUP ACM Conference on Supporting Group Work ACM2ASSETS ACM Conference on Supporting Group Work ACM3DIS ACM Conference on Designing InteractiveSystemsACM4GI Graphics Interface conference ACM 5MobiQuitous International Conference on Mobile and Ubiquitous Systems: Computing, Networking and Services Springer6PERCOM IEEE International Conference onPervasive Computing and CommunicationsIEEE7INTERACT IFIP TC13 Conference on Human-ComputerInteractionIFIP8CoopIS International Conference on Cooperative Information Systems Springer9ICMI ACM International Conference on MultimodalInteractionACM10IDC Interaction Design and Children ACM 11AVI International Working Conference on Advanced User Interfaces ACM12UIC IEEE International Conference on UbiquitousIntelligence and ComputingIEEE中国计算机学会推荐国际学术会议(前沿、交叉与综合)⼀、A类序号会议简称会议全称出版社⽹址1RTSS Real-Time Systems Symposium IEEE⼆、B类序号会议简称会议全称出版社⽹址1EMSOFT International Conference on Embedded Software ACM/IEEE/IFIP2ISMB International conference on Intelligent Systems for Molecular Biology Oxford Journals3CogSci Cognitive Science Society Annual Conference Psychology Press4RECOMB International Conference on Research in Computational Molecular Biology Springer5BIBM IEEE International Conference on Bioinformatics and Biomedicine IEEE三、C类序号会议简称会议全称出版社⽹址1AMIA American Medical Informatics Association Annual Symposium AMIA2APBC Asia Pacific Bioinformatics Conference BioMed Central3COSIT International Conference on Spatial Information Theory ACM3COSIT International Conference on Spatial Information Theory ACM。
岩土阻尼比系数标准
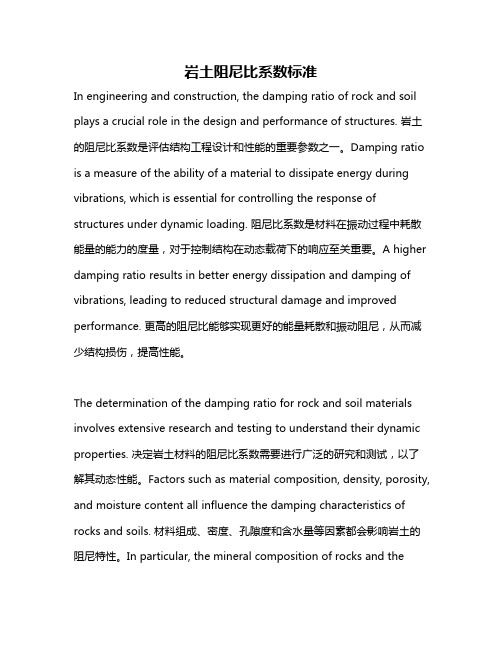
岩土阻尼比系数标准In engineering and construction, the damping ratio of rock and soil plays a crucial role in the design and performance of structures. 岩土的阻尼比系数是评估结构工程设计和性能的重要参数之一。
Damping ratio is a measure of the ability of a material to dissipate energy during vibrations, which is essential for controlling the response of structures under dynamic loading. 阻尼比系数是材料在振动过程中耗散能量的能力的度量,对于控制结构在动态载荷下的响应至关重要。
A higher damping ratio results in better energy dissipation and damping of vibrations, leading to reduced structural damage and improved performance. 更高的阻尼比能够实现更好的能量耗散和振动阻尼,从而减少结构损伤,提高性能。
The determination of the damping ratio for rock and soil materials involves extensive research and testing to understand their dynamic properties. 决定岩土材料的阻尼比系数需要进行广泛的研究和测试,以了解其动态性能。
Factors such as material composition, density, porosity, and moisture content all influence the damping characteristics of rocks and soils. 材料组成、密度、孔隙度和含水量等因素都会影响岩土的阻尼特性。
设计和参数优化的含义和流程
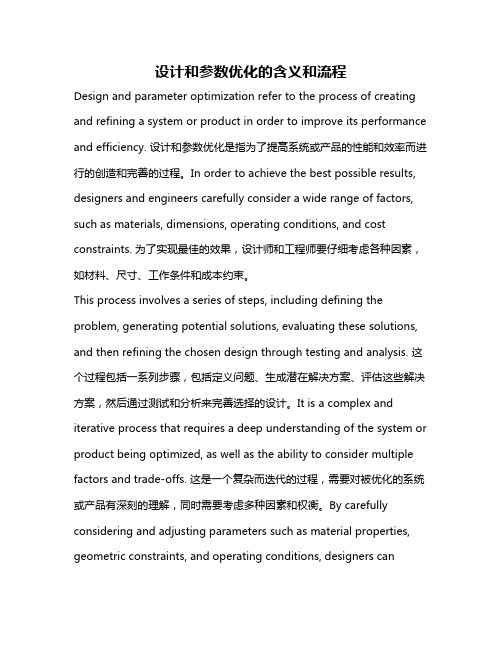
设计和参数优化的含义和流程Design and parameter optimization refer to the process of creating and refining a system or product in order to improve its performance and efficiency. 设计和参数优化是指为了提高系统或产品的性能和效率而进行的创造和完善的过程。
In order to achieve the best possible results, designers and engineers carefully consider a wide range of factors, such as materials, dimensions, operating conditions, and cost constraints. 为了实现最佳的效果,设计师和工程师要仔细考虑各种因素,如材料、尺寸、工作条件和成本约束。
This process involves a series of steps, including defining the problem, generating potential solutions, evaluating these solutions, and then refining the chosen design through testing and analysis. 这个过程包括一系列步骤,包括定义问题、生成潜在解决方案、评估这些解决方案,然后通过测试和分析来完善选择的设计。
It is a complex and iterative process that requires a deep understanding of the system or product being optimized, as well as the ability to consider multiple factors and trade-offs. 这是一个复杂而迭代的过程,需要对被优化的系统或产品有深刻的理解,同时需要考虑多种因素和权衡。
a1特种设备设计英语
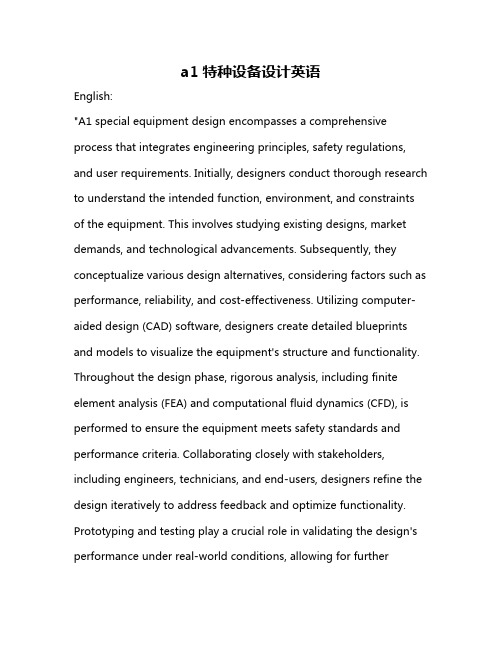
a1特种设备设计英语English:"A1 special equipment design encompasses a comprehensive process that integrates engineering principles, safety regulations, and user requirements. Initially, designers conduct thorough research to understand the intended function, environment, and constraints of the equipment. This involves studying existing designs, market demands, and technological advancements. Subsequently, they conceptualize various design alternatives, considering factors such as performance, reliability, and cost-effectiveness. Utilizing computer-aided design (CAD) software, designers create detailed blueprints and models to visualize the equipment's structure and functionality. Throughout the design phase, rigorous analysis, including finite element analysis (FEA) and computational fluid dynamics (CFD), is performed to ensure the equipment meets safety standards and performance criteria. Collaborating closely with stakeholders, including engineers, technicians, and end-users, designers refine the design iteratively to address feedback and optimize functionality. Prototyping and testing play a crucial role in validating the design's performance under real-world conditions, allowing for furtherrefinement and optimization. The final design is then documented comprehensively, including specifications, drawings, and instructions for manufacturing and operation, to ensure consistency and quality during production and usage."中文翻译:"A1特种设备设计涵盖了一个综合性的过程,将工程原理、安全法规和用户需求相互整合。
Multidisciplinary Design Optimization
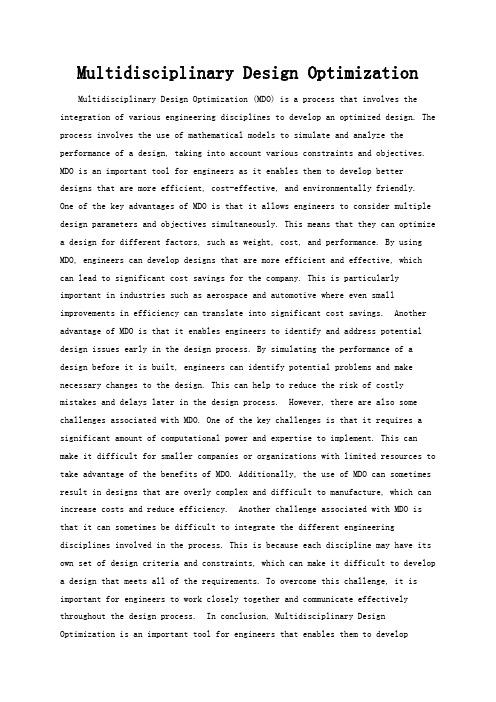
Multidisciplinary Design Optimization Multidisciplinary Design Optimization (MDO) is a process that involves the integration of various engineering disciplines to develop an optimized design. The process involves the use of mathematical models to simulate and analyze the performance of a design, taking into account various constraints and objectives. MDO is an important tool for engineers as it enables them to develop better designs that are more efficient, cost-effective, and environmentally friendly. One of the key advantages of MDO is that it allows engineers to consider multiple design parameters and objectives simultaneously. This means that they can optimize a design for different factors, such as weight, cost, and performance. By using MDO, engineers can develop designs that are more efficient and effective, which can lead to significant cost savings for the company. This is particularly important in industries such as aerospace and automotive where even small improvements in efficiency can translate into significant cost savings. Another advantage of MDO is that it enables engineers to identify and address potential design issues early in the design process. By simulating the performance of a design before it is built, engineers can identify potential problems and make necessary changes to the design. This can help to reduce the risk of costly mistakes and delays later in the design process. However, there are also some challenges associated with MDO. One of the key challenges is that it requires a significant amount of computational power and expertise to implement. This can make it difficult for smaller companies or organizations with limited resources to take advantage of the benefits of MDO. Additionally, the use of MDO can sometimes result in designs that are overly complex and difficult to manufacture, which can increase costs and reduce efficiency. Another challenge associated with MDO is that it can sometimes be difficult to integrate the different engineering disciplines involved in the process. This is because each discipline may have its own set of design criteria and constraints, which can make it difficult to develop a design that meets all of the requirements. To overcome this challenge, it is important for engineers to work closely together and communicate effectively throughout the design process. In conclusion, Multidisciplinary Design Optimization is an important tool for engineers that enables them to developbetter designs that are more efficient, cost-effective, and environmentally friendly. While there are some challenges associated with MDO, such as the needfor significant computational power and expertise, the benefits of using MDO far outweigh the challenges. By using MDO, engineers can optimize designs for multiple factors simultaneously, identify and address potential design issues early in the design process, and develop designs that are more efficient and effective. Overall, MDO is a valuable tool for engineers that can help to drive innovation and improve the quality of designs.。
船舶海洋工程面试自我介绍英文
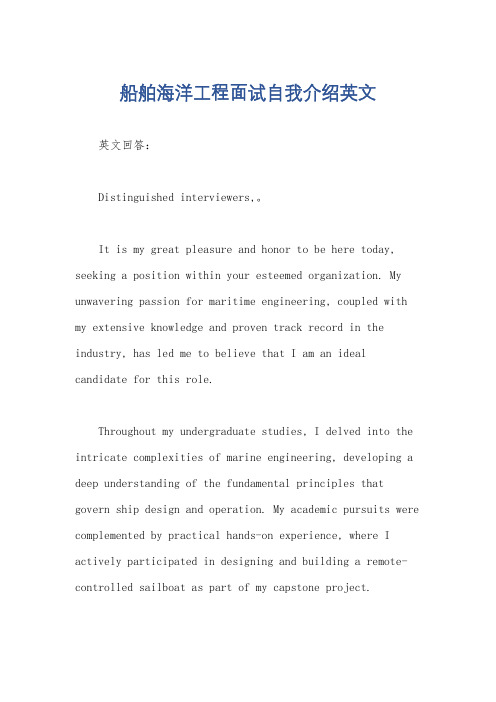
船舶海洋工程面试自我介绍英文英文回答:Distinguished interviewers,。
It is my great pleasure and honor to be here today, seeking a position within your esteemed organization. My unwavering passion for maritime engineering, coupled with my extensive knowledge and proven track record in the industry, has led me to believe that I am an ideal candidate for this role.Throughout my undergraduate studies, I delved into the intricate complexities of marine engineering, developing a deep understanding of the fundamental principles that govern ship design and operation. My academic pursuits were complemented by practical hands-on experience, where I actively participated in designing and building a remote-controlled sailboat as part of my capstone project.Upon graduating, I embarked on my professional journey at a renowned shipyard, where I quickly rose through the ranks, assuming increasingly challenging responsibilities. As a Marine Engineer, I was entrusted with overseeing the design, construction, and maintenance of various types of vessels, ranging from small fishing boats to large oceangoing cargo ships. My unwavering commitment to delivering exceptional results was recognized through my consistent track record of exceeding expectations.During my tenure at the shipyard, I developed a comprehensive understanding of ship systems, including propulsion, electrical, and auxiliary systems. I became proficient in utilizing advanced computer-aided design software and analytical tools to optimize vessel performance and ensure compliance with stringent industry regulations.Moreover, my keen interest in marine technology led me to pursue a Master's degree in Ocean Engineering, where I specialized in computational fluid dynamics and wave propagation analysis. This advanced knowledge has equippedme with the tools and expertise to tackle complex design challenges and develop innovative solutions.Beyond my technical capabilities, I am an effective communicator and a collaborative team player. I possess a natural ability to convey complex technical information clearly and concisely to both technical and non-technical audiences. My interpersonal skills and dedication to working seamlessly within a diverse team environment have been instrumental in my success.I am particularly drawn to your organization's reputation for excellence and commitment to sustainability in the maritime industry. Your pioneering work in developing environmentally friendly and fuel-efficient vessel designs aligns perfectly with my personal values and career aspirations. I am eager to contribute my knowledge and skills to your mission of shaping the future of marine engineering.Thank you for your time and consideration. I am confident that my qualifications and unwavering enthusiasmmake me a valuable asset to your team. I am eager to demonstrate my capabilities and contribute to the ongoing success of your organization.中文回答:各位尊敬的面试官,。
- 1、下载文档前请自行甄别文档内容的完整性,平台不提供额外的编辑、内容补充、找答案等附加服务。
- 2、"仅部分预览"的文档,不可在线预览部分如存在完整性等问题,可反馈申请退款(可完整预览的文档不适用该条件!)。
- 3、如文档侵犯您的权益,请联系客服反馈,我们会尽快为您处理(人工客服工作时间:9:00-18:30)。
Computational Design and Performance of the Fast Ocean Atmosphere Model,Version OneRobert Jacob1,Chad Schafer2,Ian Foster1,Michael Tobis3,andJohn Anderson31Argonne National Laboratory,Mathematics and Computer Science Division9700S.Cass Ave.,Argonne,IL60439,USA,jacob@2University of California at Berkeley,Department of Statistics367Evans Hall,Berkeley,CA94720,USA3University of Wisconsin–Madison,Space Science and Engineering Center1225W.Dayton St.,Madison,WI53706,USAAbstract.The Fast Ocean Atmosphere Model(FOAM)is a climatesystem model intended for application to climate science questions thatrequire long simulations.FOAM is a distributed-memory parallel climatemodel consisting of parallel general circulation models of the atmosphereand ocean with complete physics paramaterizations as well as sea-ice,land surface,and river transport models.FOAM’s coupling strategy waschosen for high throughput(simulated years per day).A new coupler waswritten for FOAM and some modifications were required of the compo-nent models.Performance data for FOAM on the IBM SP3and SGIOrigin2000demonstrates that it can simulate over thirty years per dayon modest numbers of processors.1IntroductionThe Earth’s climate is the long-term average of the behavior of the ocean,land surface,and sea ice.These components exchange heat,momentum,and fresh water with each other through their common interfaces.The difference in heat storage times,fluid dynamic scales,and transport/storage of water results in complex interactions between the components.To study the Earth’s climate,researchers have developed coupled models, comprising an atmospheric general circulation model(GCM),a model of the ocean general circulation,a model of the dynamic and thermodynamic prop-erties of sea ice,and a model of the temperature and composition of the land surface.Typically,each component model is a separately developed program with its own code style,language,data structures,numerical methods,and associated numerical grids.Constructing a single model from these submodels requires sev-eral design decisions about grid structure,model decomposition,andflux calcu-lations.Additionally,a“coupler”must be created to enable the subcomponents to exchange heat and other quantities.V.N.Alexandrov et al.(Eds.):ICCS2001,LNCS2073,pp.175–184,2001.c Springer-Verlag Berlin Heidelberg2001176R.Jacob et al.While many coupled climate models are used in studies that require predict-ingfine-scale details of temperature and precipitation changes,other climate sci-ence studies are more concerned with high throughput than with high resolution. For example,paleoclimate scientists wish to know how the climate responded to conditions thousands of years ago,when the earth received more solar radiation in the summer or when glaciers covered much of the northern hemisphere;and climate scientists wish to know how much low-frequency internal variability the climate system has and how it might interact with anthropogenic change.Inte-grating a climate model to even a quasi-equilibrium for the myriad of interesting paleoclimate scenarios requires hundreds of simulated years,while studying low-frequency variability requires simulations of thousands of years.Thus there is a compelling need for a climate model with high-throughput–a high number of simulated years per day.The Fast Ocean Atmosphere Model is a coupled climate model designed to meet this need.It has already been used to examine low-frequency variability and paleoclimate problems[7,11,13].This paper describes how the goal of high-throughput guided the design of FOAM and its coupler.Section2describes the component models of FOAM.The unique elements of FOAM’s software design are described in Section3.In Section4,performance data for FOAM is presented in the form of throughput per processor for various platforms.Section5briefly outlines future work on the next version of FOAM.2Component ModelsThefirst design decision made for FOAM was to use distributed-memory par-allel components.The low cost/performance ratios of distributed-memory mul-ticomputers make them the platform of choice for the kind of climate science questions FOAM is intended to address.Fortunately,FOAM was able to use several existing parallel component models,as briefly described below.(A de-tailed description of the physical equations solved by each component is beyond the scope of this paper;however,that information can be found in the cited references.)The atmospheric component of FOAM is derived from PCCM2,developed by Argonne National Laboratory,Oak Ridge National Laboratory,and the National Center for Atmospheric Research.PCCM2is a functionally equivalent version of NCAR’s CCM2in which the physics and dynamics calculations have been given a two-dimensional parallel decomposition(see Figure1a for an example). The basic equations and numerical methods used in CCM2(Eulerian-spectral elements for the dry dynamics and semi-Lagrangian transport for moisture)are described by Hack et al.[6],and the alterations necessary to implement a parallel version and its performance on various parallel platforms are described by Drake et al.[5].When CCM3[8]was released,the FOAM development team added the new physics parameterizations to the atmosphere model to create the current atmosphere component of FOAM,informally called PCCM3-UW.Computational Design and Performance177Fig.1.A depiction of how physical space is partitioned among4x4processors according to the decomposition strategy of(a)the atmosphere and(b)the ocean.The ocean component of FOAM,called OM3,is also a parallel model with a two-dimensional decomposition(Fig.1b).OM3is a z-coordinatefinite-difference model.While documentation specific to OM3is still under development,the basic equations solved are the same as those for the widely used z-coordinate Modular Ocean Model created by GFDL[4][3].However,FOAM uses a free surface as described by Killworth et al.[9]and contains numerical methods specifically chosen for their efficiency on distributed-memory parallel platforms [14].The land surface and sea-ice models in version one of FOAM are based on those of PCCM2[6]but with some important modifications.The prescribed evaporation and snow cover have been replaced with a prognostic box hydrology model from CCM1[15].Also,the effect of sea-ice creation/destruction on ocean salinity has been included.Two new software components had to be created to complete FOAM.The coupler will be described below.The other new component is a parallel river transport model.In order to prevent a constant increase in salinity of the ocean in long climate simulations,it was necessary to close the hydrologic cycle by returning precipitation that falls on land to the ocean.FOAM’s river model is a parallel implementation of concepts described by Miller et al.[12].FOAM and its individual components use the Message Passing Interface (MPI)library for communication.The land and sea-ice models do not require any communication,however,since they are implemented as one-dimensional models at each land and ocean point on the surface,respectively.3Design of the Coupled SystemIn parallel climate models,each component,especially the atmosphere,can place large demands on the communication network and CPU resources of modern parallel supercomputers.A coupled system creates additional demands on the178R.Jacob et al.bandwidth (mostly from the exchange of data between models)and on CPU re-sources (mostly from the interpolation of data between numerical grids).Design choices in FOAM were made to minimize the impact of coupling.Those choices and the reasons for them are summarized in this section.3.1Number of Numerical GridsGenerally,each model may have its own finite difference grid.FOAM’s design starts with the requirement that all surface models (land,sea ice,and ocean)use the grid of the ocean model.The intention is to eliminate a class of opera-tions in the coupler that would be required to keep track of land/ocean fraction and merge the calculated fluxes accordingly.This decision does not have a large impact on the performance of the model,but it does greatly simplify the con-ceptual picture of the coupled system.It also has implications for other design choices described below.3.2Decomposition of Component ModelsJust as each model may have its own numerical grid,each parallel model may decompose that grid in a different way over its set of MPI processes.Addition-ally,each model may have physically separate pools of processors.In order to calculate a new flux or provide to a model an internally calculated flux,such as precipitation,data that occupies the same physical space must be brought into the same physical piece of memory.The bandwidth cost of this communication will depend on how dissimilar the decompositions are and how much overlap there is of model state in memory.To minimize this cost,FOAM restricts the possible decomposition of some of the component modelsbased on a considera-tion of the time scales between components,as shown in Figure 2.In general,anFig.2.Schematic of the components of FOAM indicating which components interact on fast and slow time steps and reflecting the physical relationship between components.The parallel river model is omitted for clarity.atmosphere model has a shorter basic time step than does the ocean because ofComputational Design and Performance179 differences in the dominantfluid dynamical scales in theflows.In FOAM,the at-mosphere has a basic time step of30minutes,while the ocean uses a6-hour time step.Because of the small heat capacity of land and sea ice,their temperature structure varies with the diurnal cycle of solar heating(which is resolved by the atmosphere model),and thus they need to communicate with the atmosphere every one or two time steps.The ocean,however,with a much larger heat ca-pacity,does not have a significant diurnal cycle and needs to communicate with the atmosphere only once a day.In order to reduce the costs of communicatingfluxes,the“fast”components (Fig.2)are all given the same decomposition so that there is nearly a one-to-one correspondence in area coverage between atmosphere,land,and sea ice.The at-mosphere,land,and sea-ice models are executed sequentially on the same pool of processors so nearly all data necessary for physical coupling resides in local memory.The area coverage will not match exactly,however,because the land and sea ice are on the ocean’s numerical grid,which coincides nowhere with the atmosphere’s.Some communication is required to update points on the edges of the local rectangular regions covered by each MPI process,but this communi-cation overhead is less than what would be required if the fast components had to transfer or transpose their entire state across multiple processors every one or two time steps.The ocean model has its own decomposition and is assigned a distinct set of processors.It integrates concurrently with the atmosphere,land,and sea ice. Trial and error determines how many processors to allocate to each side to avoid blocking on munication between the ocean and atmosphere–land–sea-ice coupler is done by designating one MPI process on each side to receive all the data from the other component and redistribute it according to that component’s decomposition strategy.Since the ocean is the slow component and needs to communicate less frequently,the impact of this single-node bottleneck is minimized.3.3Flux Interpolation and CalculationOne of the basic tasks of a coupled model is to interpolate quantities from one grid to another.Calculating and interpolatingfluxes in FOAM are simplified by placing the land,sea-ice,and ocean models all onto a common grid as mentioned above.Thus,the number of grids in the model is reduced to at most two instead of possibly one per model.FOAM considers the surface of the globe as being divided into rectangles or tiles.The center of each tile is at a computational point of the model,and the four edges are halfway between each of the neighboring computational points.A third set of tiles is made by laying the ocean grid on top of the atmosphere grid, as in Fig.3a.Newfluxes are calculated on this overlap grid set of tiles.Theflux through a model tile is constructed by area-weighted averaging the appropriate pieces of the overlap tiles as shown in Fig.3b.Global conservation is assured as long as all the overlap pieces are used once and only once in the averaging calculation for each grid.180R.Jacob et al.Fig.sensible heatflux through an overlap tile can be approximated asSH=K(T S i,j,k−T A l,m),(1) where K is a constant.The triplet i,j,k indexes the pieces a given surface tile i,j is divided into(1,2,or4pieces),and l,m indexes the atmosphere tile lying above the piece.The mapping of the overlap tile indices onto each of the two grids and the area of the overlap tiles are calculated offline and stored in lookup tables for use during runtime.Once theflux at each overlap tile is known,an area-weighted aggregate is formed for each ocean and atmosphere tile in the subdomain of the coupler,as illustrated in Fig.3b.3.4FOAM’s CouplerAfter all the simplifications made with component models,FOAM’s coupler is relatively straightforward.The coupler is implemented as a subroutine of the atmosphere.The coupler performs all the communication necessary to resolve the incomplete overlapping of the local surface and atmosphere grids and calls the land,sea-ice,and river models(which are themselves subroutines of theComputational Design and Performance 181coupler).The merging of fluxes coming from separate ocean,land,and sea-ice models into a single atmospheric cell is greatly simplified by the use of single surface grid.Finally,the coupler accumulates ocean fluxes and communicates with the ocean as necessary.“Flux corrections”,which are sometimes needed to achieve a stable coupled climate,are not used in FOAM.3.5Constructing the Full ModelThe noncoincidence of the two grids presented a difficulty when combining the coupler and fast surface models with the atmosphere.In the original atmosphere model,PCCM2,all physics routines were called once for each latitude,with the surface physics occurring about two thirds of the way through the sequence.The uneven overlap of the two grids means the coupler occasionally needs two atmosphere latitudes to calculate the flux through one ocean latitude.Conse-quently,the calling tree for the PCCM2physics was split so that all the physics before the surface package could be completed for the whole globe before calling the coupler and surface models.The atmosphere resumes execution when the coupler returns.This split is still present in FOAM’s current atmosphere model PCCM3-UW.(NCAR’s CCM3,whichunlike PCCM2was designed to be part of a coupled system,contains a similar split [1].)FOAM is a single executable image.A small main program divides processors between the atmosphere-coupler-surface component and the ocean according to compile-time settings and then calls each.Some minor modifications to the atmosphere and ocean were necessary to turn them into subroutines.A summary of FOAM’s structure is shown in Fig.4.Fig.4.A schematic of FOAM’s software design.182R.Jacob et al.The atmosphere and ocean are each told by the main routine how many days to integrate.The atmosphere directs the execution of itself,the coupler,and the surface components that share the same processors.The ocean model waits to receivefluxes from the coupler and send sea surface temperatures according to a frequency set at compile time(currently every ocean time step).4Model PerformanceThe performance of FOAM and some of its components is presented in Figure5. While the atmosphere model supports many choices for truncation level of the spectral transform numerical method,the standard configuration for FOAM uses a rhomboidal truncation withfifteen wave numbers.The associated physical grid has40latitudes,48longitudes,and18levels.The standard resolution for the ocean model is a Mercator grid with128latitudes,128longitudes,and16 levels.Runs were conducted on three different platforms using configurations of5 processors(2by2for the atmosphere–land–sea-ice–coupler and1for the ocean), 9processors(2by4plus1),17processors(4by4plus1),34(4by8plus1by 2),and68processors(4by16plus2by2)processors.(The last configuration comes from atmosphere model requirements for number of local latitudes and number of processors.)Figure5shows that the model scales well over the range of configurations tested.The throughput goal for FOAM was chosen to be ten thousand times real time,that is,the ability to simulate10,000years in a single calendar year.FOAM meets this goal on a modest number of processors.Fig.5.Timings for FOAM and its components on various platforms for different num-bers of processors.Figure5and the processor configurations show how the atmosphere compo-nent dominates performance.The ocean model,despite having nearly eight timesComputational Design and Performance183 as many grid points as the atmosphere,has a much higher throughput when run-ning by itself on the same number of processors.The difference comes from the numerous,detailed physical parameterizations in the atmosphere compared with the ocean and other components.This behavior is seen in other coupled climate models also.Given the dominance of the atmosphere component on FOAM’s performance, the choice of atmosphere resolution is as important for overall throughput as the design of the coupler and the complete model.When the atmosphere model is executed by itself at a higher,more standard resolution of T42(64latitudes and 128longitudes),it is slower by a factor roughly equal to the ratio of the number of points in each grid.Figure5also shows how increases in processor speed have translated into increases in model throughput.Performance nearly doubles between the IBM SP2and IBM SP3and nearly doubles again when timed on an IBM SP3with 350MHz CPU’s(not shown).5ConclusionsThe Fast Ocean Atmosphere Model is a coupled climate model created to ad-dress climate science questions that require many simulated years of interaction but not best-possible resolution.Through a combination of a low-resolution par-allel atmosphere model,a highly efficient parallel ocean,and a software design that minimizes the effect of coupling on bandwidth and CPU usage,FOAM can simulate several decades of global climate interaction a day on modest numbers of distributed-memory parallel processors.The simulated climate is physically realistic and comparable to models with higher resolution.The rapid development of FOAM was made possible by the work done by others on the atmosphere and ocean models and also by the simplicity of land and sea-ice components.Work on the next version of FOAM will concentrate on upgrading the representation of the sea-ice and land surface to match that of the NCAR Climate System Model(CSM)[2].FOAM’s current sea ice model lacks many standard features such as ice dynamics and complex thermodynamics.In order to accommodate new models,it may be necessary to relax the conditions FOAM imposes on the land and sea-ice components.Although the resolution of FOAM’s ocean model is certainly adequate for modern sea-ice and land models, separately developed components do not usually have theflexibility to change their resolution,grid,or decomposition.A rewrite of FOAM’s coupler will be necessary to support arbitrary decompositions of land and sea-ice models;fortu-nately,that task has been made much easier by the development of new software libraries such as the Model Coupling Toolkit[10].The relationship between FOAM and larger climate modeling efforts such as CSM is complementary.FOAM uses CSM’s submodels wherever possible; adapting them to FOAM’s framework and applying them to climate questions that require more throughput than resolution.As large,institutionally sup-ported climate models increase theirflexibility and parallelism,it should be184R.Jacob et al.possible to match FOAM’s combination of throughput and simulation quality by invoking appropriate options within the larger model.Until then,FOAM development will continue.Acknowledgments:This work is supported by the Office of Biological and Environmental Research of the U.S.Department of Energy’s Office of Science under contracts W-31-109-ENG-38and DE-FG02-98ER62617.References1.Thomas L.Acker,Lawrence E.Buja,James M.Rosinski,and John E.Truesdale.User’s Guide to NCAR CCM3.NCAR Tech.Note NCAR/TN-421+IA,Natl.Cent.for Atmos.Res.,Boulder,Co.,1996.2.B.A.Boville and P.R.Gent.The NCAR climate system model,version one.J.Climate,11:1115–1130,1998.3.Kirk Bryan.A numerical method for the study of the circulation of the Worldp.Phys.,4:347–376,1969.4.M.D.Cox.A Primitive Equation three-dimensional model of the ocean.TechnicalReport GFDL Ocean Group Tech.Rep.1,GFDL,Princeton,NJ,1984.5.J.Drake,I.Foster,J.Michalakes,Brian Toonen,and Pat Worley.Design andperformance of a scalable parallel community climate model.Parallel Computing, 21(10):1571–1591,October1995.6.James J.Hack,Byron A.Boville,Bruce P.Briegleb,Jeffrey T.Kiehl,Philip J.Rasch,and David L.Williamson.Description of the NCAR Community Climate Model(CCM2).NCAR Tech.Note NCAR/TN-382+STR,Natl.Cent.for Atmos.Res.,Boulder,Co.,1993.7.Robert Jacob.Low Frequency Variability in a Simulated Atmosphere Ocean System.PhD thesis,University of Wisconsin-Madison,1997.8.Jeffrey T.Kiehl,James J.Hack,Gordon B.Bonan,Byron A.Boville,Bruce P.Briegleb,David L.Williamson,and Philip J.Rasch.Description of the NCAR Community Climate Model(CCM3).NCAR Tech.Note NCAR/TN-420+STR, Natl.Cent.for Atmos.Res.,Boulder,Co.,1996.9.P.D.Killworth,D.Stainforth,D.J.Webb,and S.M.Patterson.The developmentof a free-surface Bryan-Cox-Semtner ocean model.J.Phys.Oceanogr.,21:1333–1348,1991.10.Jay Larson and Robert Jacob.A message-passing parallel Model Coupling Toolkit(MCT).in prep.,2001.11.Zhengyu Liu,John Kutzbach,and Lixin Wu.Modeling climate shift of El-Ni˜n ovariability in the holocene.Geophy.Res.Lett.,27:2265–2268,2000.12.James ler,Gary L.Russell,and Guilherme Caliri.Continental-scale riverflow in climate models.J.Climate,7:914–928,1994.13.Chris Poulsen,Raymond T.Pierrehumbert,and Robert L.Jacob.Impact of oceandynamics on the simulation of the neoproterozoic“snowball”earth.Geophy.Res.Lett.in press,2001.14.Michael Tobis.Effect of Slowed Barotropic Dynamics in Parallel Ocean ClimateModels.PhD thesis,University of Wisconsin-Madison,1996.15.David L.Williamson,Jeffrey T.Kiehl,V.Ramanathan,Robert E.Dickinson,andJames J.Hack.Description of NCAR Community Climate Model(CCM1).NCAR Tech.Note NCAR/TN-285+STR,Natl.Cent.for Atmos.Res.,Boulder,Co.,1987.。