chapter 6 modulation techniques for mobile radio(16-04)
移动通信课程 Modulation Techniques for
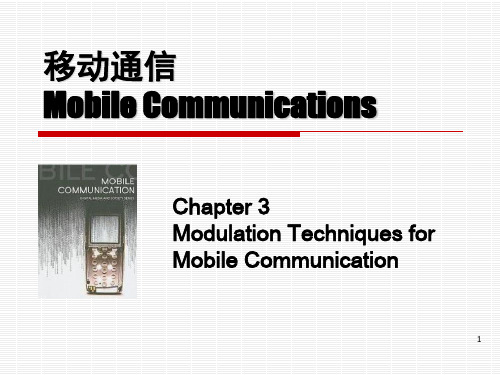
7
Amplitude Modulation
The amplitude of high-carrier signal is varied according to the instantaneous amplitude of the modulating message signal m(t).
移动通信 Mobile Communications
Chapter 3 Modulation Techniques for Mobile Communication
1
2
What is modulation
为什么需要调制?
数字基带信号具有丰
Modulation
is
the
process
of
富的低频分量
eOOK
(t
)
A 0
cos
ct 以概率P发送“1” 以概率1-P发送“0”
1 00 1 S( t )
Expression
Modulating Signal
e2ASK (t ) st cosct
Carrier Signal
Carrier Signal
2ASK
NRZ(单极性全占空)信号
s( t ) an g( t nTs ) n
More secure link
13
Digital Modulation
The modulating signal is represented as a time-sequence of symbols or pulses.
Each symbol has m finite states: That means each symbol carries n bits of information where n = log2m bits/symbol.
E第6章 PWM控制技术
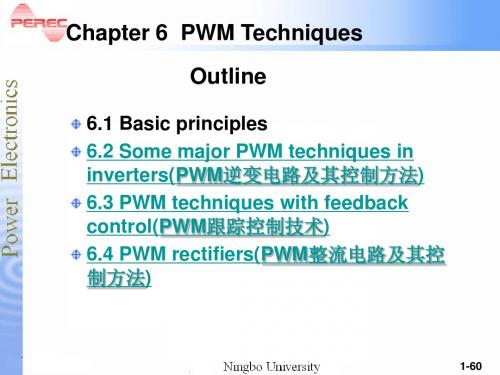
d
O
-
wt
U
d
7-60
Basic principles of PWM
等幅PWM波
Ud
O
- Ud
wt
输入电源是恒定直流 第3章的直流斩波电路 6.2节的PWM逆变电路 6.4节的PWM整流电路
U
不等幅PWM波
输入电源是交流或不是恒定 t 的直流 ω 4.1节的斩控式交流调压电路 4.4节的矩阵式变频电路
17-60
19-60
Modulation and Triangular-wave natural sampling Bi-polar PWM in single-phase VSI
u
ur
uc
O
wt
uo Ud O -U d
u of
uo
wt
Bi-polar sampling is used to realize bi-polar PWM.
1-60
Chapter 6 PWM Techniques
PWM (Pulse Width Modulation):通过对一系列脉冲的宽度进行调制,来 等效的获得所需要的波形(含形状和幅值)。 The most widely used control technique in power electronics(第3章 直流斩波电路采用的就PWM技术;第4章的4.1斩控式调压电路和4.4矩阵 式变频电路,第5章逆变电路都涉及到了。)
uc ur ur 正半周,V1 保持通,V2 保持 u 断。 当 ur>uc 时 使 V4 通 , V3 断 , O wt uo=Ud 。 当 ur<uc 时 使 V4 断 , V3 通 , uo uo=0 。 uo Ud u of 这样就在负载端得到了SPWM 表示uo 波形u0。 的基波O wt ur负半周,请同学们自己分析。 分量 上述PWM波只在单个极性范围 -U d 内变化---- Uni-polar PWM 。 图6-5 单极性PWM控制方式波形
dpwm计算
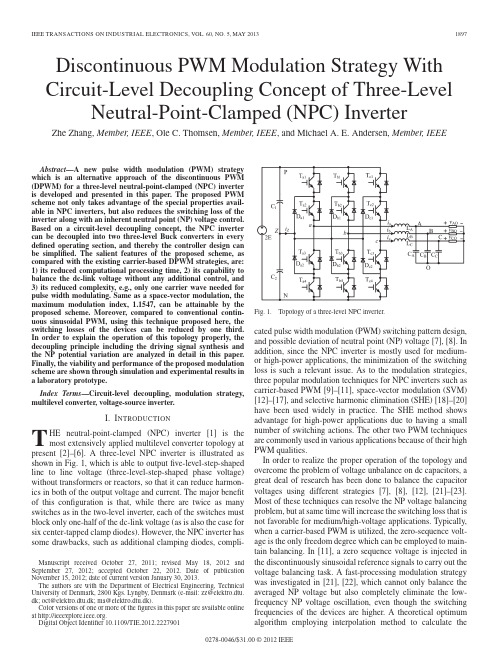
1897
Discontinuous PWM Modulation Strategy With Circuit-Level Decoupling Concept of Three-Level
Fig. 1. Topology of a three-level NPC inverter.
cated pulse width modulation (PWM) switching pattern design, and possible deviation of neutral point (NP) voltage [7], [8]. In addition, since the NPC inverter is mostly used for mediumor high-power applications, the minimization of the switching loss is such a relevant issue. As to the modulation strategies, three popular modulation techniques for NPC inverters such as carrier-based PWM [9]–[11], space-vector modulation (SVM) [12]–[17], and selective harmonic elimination (SHE) [18]–[20] have been used widely in practice. The SHE method shows advantage for high-power applications due to having a small number of switching actions. The other two PWM techniques are commonly used in various applications because of their high PWM qualities.
数字无线通信系统中的调制(英文)
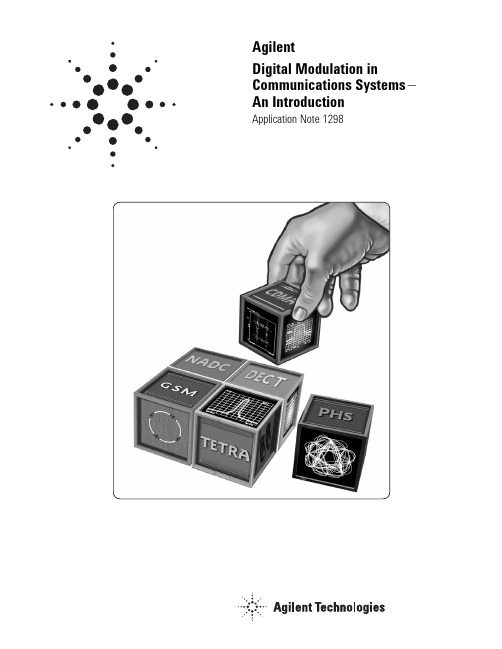
AgilentDigital Modulation in Communications Systems—An IntroductionApplication Note 1298This application note introduces the concepts of digital modulation used in many communications systems today. Emphasis is placed on explaining the tradeoffs that are made to optimize efficiencies in system design.Most communications systems fall into one of three categories: bandwidth efficient, power efficient, or cost efficient. Bandwidth efficiency describes the ability of a modulation scheme to accommodate data within a limited bandwidth. Power efficiency describes the ability of the system to reliably send information at the lowest practical power level.In most systems, there is a high priority on band-width efficiency. The parameter to be optimized depends on the demands of the particular system, as can be seen in the following two examples.For designers of digital terrestrial microwave radios, their highest priority is good bandwidth efficiency with low bit-error-rate. They have plenty of power available and are not concerned with power efficiency. They are not especially con-cerned with receiver cost or complexity because they do not have to build large numbers of them. On the other hand, designers of hand-held cellular phones put a high priority on power efficiency because these phones need to run on a battery. Cost is also a high priority because cellular phones must be low-cost to encourage more users. Accord-ingly, these systems sacrifice some bandwidth efficiency to get power and cost efficiency. Every time one of these efficiency parameters (bandwidth, power, or cost) is increased, another one decreases, becomes more complex, or does not perform well in a poor environment. Cost is a dom-inant system priority. Low-cost radios will always be in demand. In the past, it was possible to make a radio low-cost by sacrificing power and band-width efficiency. This is no longer possible. The radio spectrum is very valuable and operators who do not use the spectrum efficiently could lose their existing licenses or lose out in the competition for new ones. These are the tradeoffs that must be considered in digital RF communications design. This application note covers•the reasons for the move to digital modulation;•how information is modulated onto in-phase (I) and quadrature (Q) signals;•different types of digital modulation;•filtering techniques to conserve bandwidth; •ways of looking at digitally modulated signals;•multiplexing techniques used to share the transmission channel;•how a digital transmitter and receiver work;•measurements on digital RF communications systems;•an overview table with key specifications for the major digital communications systems; and •a glossary of terms used in digital RF communi-cations.These concepts form the building blocks of any communications system. If you understand the building blocks, then you will be able to under-stand how any communications system, present or future, works.Introduction25 5 677 7 8 8 9 10 10 1112 12 12 13 14 14 15 15 16 17 18 19 20 21 22 22 23 23 24 25 26 27 28 29 29 30 311. Why Digital Modulation?1.1 Trading off simplicity and bandwidth1.2 Industry trends2. Using I/Q Modulation (Amplitude and Phase Control) to Convey Information2.1 Transmitting information2.2 Signal characteristics that can be modified2.3 Polar display—magnitude and phase representedtogether2.4 Signal changes or modifications in polar form2.5 I/Q formats2.6 I and Q in a radio transmitter2.7 I and Q in a radio receiver2.8 Why use I and Q?3. Digital Modulation Types and Relative Efficiencies3.1 Applications3.1.1 Bit rate and symbol rate3.1.2 Spectrum (bandwidth) requirements3.1.3 Symbol clock3.2 Phase Shift Keying (PSK)3.3 Frequency Shift Keying3.4 Minimum Shift Keying (MSK)3.5 Quadrature Amplitude Modulation (QAM)3.6 Theoretical bandwidth efficiency limits3.7 Spectral efficiency examples in practical radios3.8 I/Q offset modulation3.9 Differential modulation3.10 Constant amplitude modulation4. Filtering4.1 Nyquist or raised cosine filter4.2 Transmitter-receiver matched filters4.3 Gaussian filter4.4 Filter bandwidth parameter alpha4.5 Filter bandwidth effects4.6 Chebyshev equiripple FIR (finite impulse response) filter4.7 Spectral efficiency versus power consumption5. Different Ways of Looking at a Digitally Modulated Signal Time and Frequency Domain View5.1 Power and frequency view5.2 Constellation diagrams5.3 Eye diagrams5.4 Trellis diagramsTable of Contents332 32 32 33 33 34 3435 35 3637 37 37 38 38 39 39 39 40 41 41 42 434344466. Sharing the Channel6.1 Multiplexing—frequency6.2 Multiplexing—time6.3 Multiplexing—code6.4 Multiplexing—geography6.5 Combining multiplexing modes6.6 Penetration versus efficiency7. How Digital Transmitters and Receivers Work7.1 A digital communications transmitter7.2 A digital communications receiver8. Measurements on Digital RF Communications Systems 8.1 Power measurements8.1.1 Adjacent Channel Power8.2 Frequency measurements8.2.1 Occupied bandwidth8.3 Timing measurements8.4 Modulation accuracy8.5 Understanding Error Vector Magnitude (EVM)8.6 Troubleshooting with error vector measurements8.7 Magnitude versus phase error8.8 I/Q phase error versus time8.9 Error Vector Magnitude versus time8.10 Error spectrum (EVM versus frequency)9. Summary10. Overview of Communications Systems11. Glossary of TermsTable of Contents (continued)4The move to digital modulation provides more information capacity, compatibility with digital data services, higher data security, better quality communications, and quicker system availability. Developers of communications systems face these constraints:•available bandwidth•permissible power•inherent noise level of the systemThe RF spectrum must be shared, yet every day there are more users for that spectrum as demand for communications services increases. Digital modulation schemes have greater capacity to con-vey large amounts of information than analog mod-ulation schemes. 1.1 Trading off simplicity and bandwidthThere is a fundamental tradeoff in communication systems. Simple hardware can be used in transmit-ters and receivers to communicate information. However, this uses a lot of spectrum which limits the number of users. Alternatively, more complex transmitters and receivers can be used to transmit the same information over less bandwidth. The transition to more and more spectrally efficient transmission techniques requires more and more complex hardware. Complex hardware is difficult to design, test, and build. This tradeoff exists whether communication is over air or wire, analog or digital.Figure 1. The Fundamental Tradeoff1. Why Digital Modulation?51.2 Industry trendsOver the past few years a major transition has occurred from simple analog Amplitude Mod-ulation (AM) and Frequency/Phase Modulation (FM/PM) to new digital modulation techniques. Examples of digital modulation include•QPSK (Quadrature Phase Shift Keying)•FSK (Frequency Shift Keying)•MSK (Minimum Shift Keying)•QAM (Quadrature Amplitude Modulation) Another layer of complexity in many new systems is multiplexing. Two principal types of multiplex-ing (or “multiple access”) are TDMA (Time Division Multiple Access) and CDMA (Code Division Multiple Access). These are two different ways to add diversity to signals allowing different signals to be separated from one another.Figure 2. Trends in the Industry62.1 Transmitting informationTo transmit a signal over the air, there are three main steps:1.A pure carrier is generated at the transmitter.2.The carrier is modulated with the informationto be transmitted. Any reliably detectablechange in signal characteristics can carryinformation.3.At the receiver the signal modifications orchanges are detected and demodulated.2.2 Signal characteristics that can be modified There are only three characteristics of a signal that can be changed over time: amplitude, phase, or fre-quency. However, phase and frequency are just dif-ferent ways to view or measure the same signal change. In AM, the amplitude of a high-frequency carrier signal is varied in proportion to the instantaneous amplitude of the modulating message signal.Frequency Modulation (FM) is the most popular analog modulation technique used in mobile com-munications systems. In FM, the amplitude of the modulating carrier is kept constant while its fre-quency is varied by the modulating message signal.Amplitude and phase can be modulated simultane-ously and separately, but this is difficult to gener-ate, and especially difficult to detect. Instead, in practical systems the signal is separated into another set of independent components: I(In-phase) and Q(Quadrature). These components are orthogonal and do not interfere with each other.Figure 3. Transmitting Information (Analog or Digital)Figure 4. Signal Characteristics to Modify2. Using I/Q Modulation to Convey Information72.3 Polar display—magnitude and phase repre-sented togetherA simple way to view amplitude and phase is with the polar diagram. The carrier becomes a frequency and phase reference and the signal is interpreted relative to the carrier. The signal can be expressed in polar form as a magnitude and a phase. The phase is relative to a reference signal, the carrier in most communication systems. The magnitude is either an absolute or relative value. Both are used in digital communication systems. Polar diagrams are the basis of many displays used in digital com-munications, although it is common to describe the signal vector by its rectangular coordinates of I (In-phase) and Q(Quadrature).2.4 Signal changes or modifications inpolar formFigure 6 shows different forms of modulation in polar form. Magnitude is represented as the dis-tance from the center and phase is represented as the angle.Amplitude modulation (AM) changes only the magnitude of the signal. Phase modulation (PM) changes only the phase of the signal. Amplitude and phase modulation can be used together. Frequency modulation (FM) looks similar to phase modulation, though frequency is the controlled parameter, rather than relative phase.Figure 6. Signal Changes or Modifications8One example of the difficulties in RF design can be illustrated with simple amplitude modulation. Generating AM with no associated angular modula-tion should result in a straight line on a polar display. This line should run from the origin to some peak radius or amplitude value. In practice, however, the line is not straight. The amplitude modulation itself often can cause a small amount of unwanted phase modulation. The result is a curved line. It could also be a loop if there is any hysteresis in the system transfer function. Some amount of this distortion is inevitable in any sys-tem where modulation causes amplitude changes. Therefore, the degree of effective amplitude modu-lation in a system will affect some distortion parameters.2.5 I/Q formatsIn digital communications, modulation is often expressed in terms of I and Q. This is a rectangular representation of the polar diagram. On a polar diagram, the I axis lies on the zero degree phase reference, and the Q axis is rotated by 90 degrees. The signal vector’s projection onto the I axis is its “I” component and the projection onto the Q axisis its “Q” component.Figure 7. “I-Q” Format92.6 I and Q in a radio transmitterI/Q diagrams are particularly useful because they mirror the way most digital communications sig-nals are created using an I/Q modulator. In the transmitter, I and Q signals are mixed with the same local oscillator (LO). A 90 degree phase shifter is placed in one of the LO paths. Signals that are separated by 90 degrees are also known as being orthogonal to each other or in quadrature. Signals that are in quadrature do not interfere with each other. They are two independent compo-nents of the signal. When recombined, they are summed to a composite output signal. There are two independent signals in I and Q that can be sent and received with simple circuits. This simpli-fies the design of digital radios. The main advan-tage of I/Q modulation is the symmetric ease of combining independent signal components into a single composite signal and later splitting such a composite signal into its independent component parts. 2.7 I and Q in a radio receiverThe composite signal with magnitude and phase (or I and Q) information arrives at the receiver input. The input signal is mixed with the local oscillator signal at the carrier frequency in two forms. One is at an arbitrary zero phase. The other has a 90 degree phase shift. The composite input signal (in terms of magnitude and phase) is thus broken into an in-phase, I, and a quadrature, Q, component. These two components of the signal are independent and orthogonal. One can be changed without affecting the other. Normally, information cannot be plotted in a polar format and reinterpreted as rectangular values without doing a polar-to-rectangular conversion. This con-version is exactly what is done by the in-phase and quadrature mixing processes in a digital radio. A local oscillator, phase shifter, and two mixers can perform the conversion accurately and efficiently.Figure 8. I and Q in a Practical Radio Transmitter Figure 9. I and Q in a Radio Receiver102.8 Why use I and Q?Digital modulation is easy to accomplish with I/Q modulators. Most digital modulation maps the data to a number of discrete points on the I/Q plane. These are known as constellation points. As the sig-nal moves from one point to another, simultaneous amplitude and phase modulation usually results. To accomplish this with an amplitude modulator and a phase modulator is difficult and complex. It is also impossible with a conventional phase modu-lator. The signal may, in principle, circle the origin in one direction forever, necessitating infinite phase shifting capability. Alternatively, simultaneous AM and Phase Modulation is easy with an I/Q modulator. The I and Q control signals are bounded, but infi-nite phase wrap is possible by properly phasing the I and Q signals.This section covers the main digital modulation formats, their main applications, relative spectral efficiencies, and some variations of the main modulation types as used in practical systems. Fortunately, there are a limited number of modula-tion types which form the building blocks of any system.3.1 ApplicationsThe table below covers the applications for differ-ent modulation formats in both wireless communi-cations and video. Although this note focuses on wireless communica-tions, video applications have also been included in the table for completeness and because of their similarity to other wireless communications.3.1.1 Bit rate and symbol rateTo understand and compare different modulation format efficiencies, it is important to first under-stand the difference between bit rate and symbol rate. The signal bandwidth for the communications channel needed depends on the symbol rate, not on the bit rate.Symbol rate =bit ratethe number of bits transmitted with each symbol 3. Digital Modulation Types and Relative EfficienciesBit rate is the frequency of a system bit stream. Take, for example, a radio with an 8 bit sampler, sampling at 10 kHz for voice. The bit rate, the basic bit stream rate in the radio, would be eight bits multiplied by 10K samples per second, or 80 Kbits per second. (For the moment we will ignore the extra bits required for synchronization, error correction, etc.)Figure 10 is an example of a state diagram of a Quadrature Phase Shift Keying (QPSK) signal. The states can be mapped to zeros and ones. This is a common mapping, but it is not the only one. Any mapping can be used.The symbol rate is the bit rate divided by the num-ber of bits that can be transmitted with each sym-bol. If one bit is transmitted per symbol, as with BPSK, then the symbol rate would be the same as the bit rate of 80 Kbits per second. If two bits are transmitted per symbol, as in QPSK, then the sym-bol rate would be half of the bit rate or 40 Kbits per second. Symbol rate is sometimes called baud rate. Note that baud rate is not the same as bit rate. These terms are often confused. If more bits can be sent with each symbol, then the same amount of data can be sent in a narrower spec-trum. This is why modulation formats that are more complex and use a higher number of states can send the same information over a narrower piece of the RF spectrum.3.1.2 Spectrum (bandwidth) requirementsAn example of how symbol rate influences spec-trum requirements can be seen in eight-state Phase Shift Keying (8PSK). It is a variation of PSK. There are eight possible states that the signal can transi-tion to at any time. The phase of the signal can take any of eight values at any symbol time. Since 23= 8, there are three bits per symbol. This means the symbol rate is one third of the bit rate. This is relatively easy to decode.Figure 10. Bit Rate and Symbol Rate Figure 11. Spectrum Requirements3.1.3 Symbol ClockThe symbol clock represents the frequency and exact timing of the transmission of the individual symbols. At the symbol clock transitions, the trans-mitted carrier is at the correct I/Q(or magnitude/ phase) value to represent a specific symbol (a specific point in the constellation).3.2 Phase Shift KeyingOne of the simplest forms of digital modulation is binary or Bi-Phase Shift Keying (BPSK). One appli-cation where this is used is for deep space teleme-try. The phase of a constant amplitude carrier sig-nal moves between zero and 180 degrees. On an I and Q diagram, the I state has two different values. There are two possible locations in the state dia-gram, so a binary one or zero can be sent. The symbol rate is one bit per symbol.A more common type of phase modulation is Quadrature Phase Shift Keying (QPSK). It is used extensively in applications including CDMA (Code Division Multiple Access) cellular service, wireless local loop, Iridium (a voice/data satellite system) and DVB-S (Digital Video Broadcasting — Satellite). Quadrature means that the signal shifts between phase states which are separated by 90 degrees. The signal shifts in increments of 90 degrees from 45 to 135, –45, or –135 degrees. These points are chosen as they can be easily implemented using an I/Q modulator. Only two I values and two Q values are needed and this gives two bits per symbol. There are four states because 22= 4. It is therefore a more bandwidth-efficient type of modulation than BPSK, potentially twice as efficient.Figure 12. Phase Shift Keying3.3 Frequency Shift KeyingFrequency modulation and phase modulation are closely related. A static frequency shift of +1 Hz means that the phase is constantly advancing at the rate of 360 degrees per second (2 πrad/sec), relative to the phase of the unshifted signal.FSK (Frequency Shift Keying) is used in many applications including cordless and paging sys-tems. Some of the cordless systems include DECT (Digital Enhanced Cordless Telephone) and CT2 (Cordless Telephone 2).In FSK, the frequency of the carrier is changed as a function of the modulating signal (data) being transmitted. Amplitude remains unchanged. In binary FSK (BFSK or 2FSK), a “1” is represented by one frequency and a “0” is represented by another frequency.3.4 Minimum Shift KeyingSince a frequency shift produces an advancing or retarding phase, frequency shifts can be detected by sampling phase at each symbol period. Phase shifts of (2N + 1) π/2radians are easily detected with an I/Q demodulator. At even numbered sym-bols, the polarity of the I channel conveys the transmitted data, while at odd numbered symbols the polarity of the Q channel conveys the data. This orthogonality between I and Q simplifies detection algorithms and hence reduces power con-sumption in a mobile receiver. The minimum fre-quency shift which yields orthogonality of I and Q is that which results in a phase shift of ±π/2radi-ans per symbol (90 degrees per symbol). FSK with this deviation is called MSK (Minimum Shift Keying). The deviation must be accurate in order to generate repeatable 90 degree phase shifts. MSK is used in the GSM (Global System for Mobile Communications) cellular standard. A phase shift of +90 degrees represents a data bit equal to “1,”while –90 degrees represents a “0.” The peak-to-peak frequency shift of an MSK signal is equal to one-half of the bit rate.FSK and MSK produce constant envelope carrier signals, which have no amplitude variations. This is a desirable characteristic for improving the power efficiency of transmitters. Amplitude varia-tions can exercise nonlinearities in an amplifier’s amplitude-transfer function, generating spectral regrowth, a component of adjacent channel power. Therefore, more efficient amplifiers (which tend to be less linear) can be used with constant-envelope signals, reducing power consumption.Figure 13. Frequency Shift KeyingMSK has a narrower spectrum than wider devia-tion forms of FSK. The width of the spectrum is also influenced by the waveforms causing the fre-quency shift. If those waveforms have fast transi-tions or a high slew rate, then the spectrumof the transmitter will be broad. In practice, the waveforms are filtered with a Gaussian filter, resulting in a narrow spectrum. In addition, the Gaussian filter has no time-domain overshoot, which would broaden the spectrum by increasing the peak deviation. MSK with a Gaussian filter is termed GMSK (Gaussian MSK).3.5 Quadrature Amplitude ModulationAnother member of the digital modulation family is Quadrature Amplitude Modulation (QAM). QAM is used in applications including microwave digital radio, DVB-C (Digital Video Broadcasting—Cable), and modems.In 16-state Quadrature Amplitude Modulation (16QAM), there are four I values and four Q values. This results in a total of 16 possible states for the signal. It can transition from any state to any other state at every symbol time. Since 16 = 24, four bits per symbol can be sent. This consists of two bits for I and two bits for Q. The symbol rate is one fourth of the bit rate. So this modulation format produces a more spectrally efficient transmission. It is more efficient than BPSK, QPSK, or 8PSK. Note that QPSK is the same as 4QAM.Another variation is 32QAM. In this case there are six I values and six Q values resulting in a total of 36 possible states (6x6=36). This is too many states for a power of two (the closest power of two is 32). So the four corner symbol states, which take the most power to transmit, are omitted. This reduces the amount of peak power the transmitter has to generate. Since 25= 32, there are five bits per sym-bol and the symbol rate is one fifth of the bit rate. The current practical limits are approximately256QAM, though work is underway to extend the limits to 512 or 1024 QAM. A 256QAM system uses 16 I-values and 16 Q-values, giving 256 possible states. Since 28= 256, each symbol can represent eight bits. A 256QAM signal that can send eight bits per symbol is very spectrally efficient. However, the symbols are very close together and are thus more subject to errors due to noise and distortion. Such a signal may have to be transmit-ted with extra power (to effectively spread the symbols out more) and this reduces power efficiency as compared to simpler schemes.Figure 14. Quadrature Amplitude ModulationCompare the bandwidth efficiency when using256QAM versus BPSK modulation in the radio example in section 3.1.1 (which uses an eight-bit sampler sampling at 10 kHz for voice). BPSK uses80 Ksymbols-per-second sending 1 bit per symbol.A system using 256QAM sends eight bits per sym-bol so the symbol rate would be 10 Ksymbols per second. A 256QAM system enables the same amount of information to be sent as BPSK using only one eighth of the bandwidth. It is eight times more bandwidth efficient. However, there is a tradeoff. The radio becomes more complex and is more susceptible to errors caused by noise and dis-tortion. Error rates of higher-order QAM systems such as this degrade more rapidly than QPSK as noise or interference is introduced. A measureof this degradation would be a higher Bit Error Rate (BER).In any digital modulation system, if the input sig-nal is distorted or severely attenuated the receiver will eventually lose symbol lock completely. If the receiver can no longer recover the symbol clock, it cannot demodulate the signal or recover any infor-mation. With less degradation, the symbol clock can be recovered, but it is noisy, and the symbol locations themselves are noisy. In some cases, a symbol will fall far enough away from its intended position that it will cross over to an adjacent posi-tion. The I and Q level detectors used in the demodulator would misinterpret such a symbol as being in the wrong location, causing bit errors. QPSK is not as efficient, but the states are much farther apart and the system can tolerate a lot more noise before suffering symbol errors. QPSK has no intermediate states between the four corner-symbol locations, so there is less opportunity for the demodulator to misinterpret symbols. QPSK requires less transmitter power than QAM to achieve the same bit error rate.3.6 Theoretical bandwidth efficiency limits Bandwidth efficiency describes how efficiently the allocated bandwidth is utilized or the ability of a modulation scheme to accommodate data, within a limited bandwidth. The table below shows the theoretical bandwidth efficiency limits for the main modulation types. Note that these figures cannot actually be achieved in practical radios since they require perfect modulators, demodula-tors, filter, and transmission paths.If the radio had a perfect (rectangular in the fre-quency domain) filter, then the occupied band-width could be made equal to the symbol rate.Techniques for maximizing spectral efficiency include the following:•Relate the data rate to the frequency shift (as in GSM).•Use premodulation filtering to reduce the occupied bandwidth. Raised cosine filters,as used in NADC, PDC, and PHS, give thebest spectral efficiency.•Restrict the types of transitions.Modulation Theoretical bandwidthformat efficiencylimitsMSK 1bit/second/HzBPSK 1bit/second/HzQPSK 2bits/second/Hz8PSK 3bits/second/Hz16 QAM 4 bits/second/Hz32 QAM 5 bits/second/Hz64 QAM 6 bits/second/Hz256 QAM 8 bits/second/HzEffects of going through the originTake, for example, a QPSK signal where the normalized value changes from 1, 1 to –1, –1. When changing simulta-neously from I and Q values of +1 to I and Q values of –1, the signal trajectory goes through the origin (the I/Q value of 0,0). The origin represents 0 carrier magnitude. A value of 0 magnitude indicates that the carrier amplitude is 0 for a moment.Not all transitions in QPSK result in a trajectory that goes through the origin. If I changes value but Q does not (or vice-versa) the carrier amplitude changes a little, but it does not go through zero. Therefore some symbol transi-tions will result in a small amplitude variation, while others will result in a very large amplitude variation. The clock-recovery circuit in the receiver must deal with this ampli-tude variation uncertainty if it uses amplitude variations to align the receiver clock with the transmitter clock. Spectral regrowth does not automatically result from these trajectories that pass through or near the origin. If the amplifier and associated circuits are perfectly linear, the spectrum (spectral occupancy or occupied bandwidth) will be unchanged. The problem lies in nonlinearities in the circuits.A signal which changes amplitude over a very large range will exercise these nonlinearities to the fullest extent. These nonlinearities will cause distortion products. In con-tinuously modulated systems they will cause “spectral regrowth” or wider modulation sidebands (a phenomenon related to intermodulation distortion). Another term which is sometimes used in this context is “spectral splatter.”However this is a term that is more correctly used in asso-ciation with the increase in the bandwidth of a signal caused by pulsing on and off.3.7 Spectral efficiency examples inpractical radiosThe following examples indicate spectral efficien-cies that are achieved in some practical radio systems.The TDMA version of the North American Digital Cellular (NADC) system, achieves a 48 Kbits-per-second data rate over a 30 kHz bandwidth or 1.6 bits per second per Hz. It is a π/4 DQPSK based system and transmits two bits per symbol. The theoretical efficiency would be two bits per second per Hz and in practice it is 1.6 bits per second per Hz.Another example is a microwave digital radio using 16QAM. This kind of signal is more susceptible to noise and distortion than something simpler such as QPSK. This type of signal is usually sent over a direct line-of-sight microwave link or over a wire where there is very little noise and interference. In this microwave-digital-radio example the bit rate is 140 Mbits per second over a very wide bandwidth of 52.5 MHz. The spectral efficiency is 2.7 bits per second per Hz. To implement this, it takes a very clear line-of-sight transmission path and a precise and optimized high-power transceiver.。
无线通信原理和应用习题课2
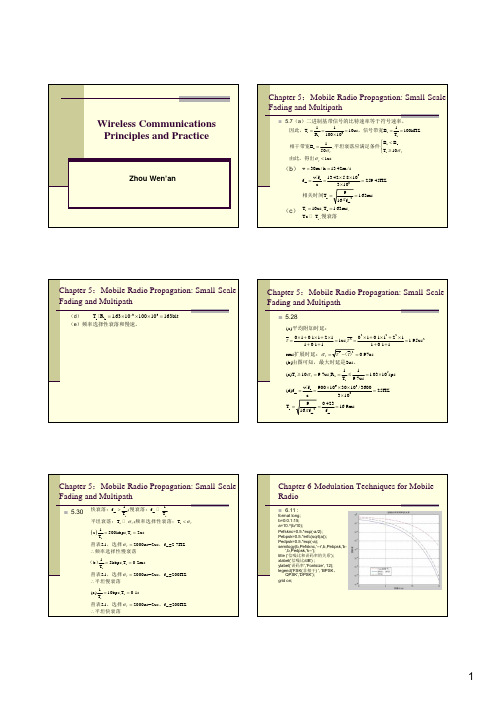
则
Eb W /R E N = 所以 W= b ( − 1) ⋅ R ⋅ a = 16.8( Mchips / s ) N 0 ( N / 3 − 1) ⋅ a N0 3
2
祝大家: 期末顺利! 天天开心!
3
− P −1 ( x ) l n (1 − y ) b):与式(7.58)类似 得到: ∴ γ
0
=
而GSM每信道发送数据速率为270.8333kbps,约是0.136C
y = [1 − e
−
p −1 ( x )
γ0
]M
Chapter7 Equalization, Diversity, and Channel Coding
6.11 :
format long; b=0:0.1:15; a=10.^(b/10); Pefsknc=0.5.*exp(-a/2); Pebpsk=0.5.*erfc(sqrt(a)); Pedpsk=0.5.*exp(-a); semilogy(b,Pefsknc,'--r',b,Pebpsk,'b',b,Pedpsk,'k--'); title ('信噪比和误码率的关系'); xlabel('信噪比/dB') ; ylabel('误码率','Fontsize', 12); legend('FSK(非相干) ', 'BPSK, QPSK','DPSK'); grid on;
Chapter 9:Multiple Access Techniques for Wireless Communications
9.7 a) 发送一个分组的时间τ
无线通信英文版教学设计 (2)

无线通信英文版教学设计1. IntroductionWireless communication is the exchange of information between two or more devices without the use of cables or wires. Wireless communication is an essential part of modern life and has transformed the way we communicate. This course is designed to provide students with a solid foundation in wireless communication principles, practices, and technologies.2. Course ObjectivesThe objectives of this course are: - To introduce the fundamental principles of wireless communication. - To expln the different types of wireless communication systems and their applications. - To teach the basic concepts of radio frequency (RF) technology and wireless network architecture. - To discuss the various communication standards that govern wireless communication. - To provide hands-on experience in building wireless communication systems.3. Course OutlineThe course will cover the following topics:Week 1: Introduction to Wireless Communication•Overview of wireless communication•Types of wireless communication systems•Applications of wireless communicationWeek 2: Basic Concepts of Radio Frequency (RF) Technology•RF waves and signals•Modulation techniques•Demodulation techniquesWeek 3: Wireless Network Architecture•Wireless network topologies•Wireless access points and routers•Wireless network securityWeek 4: Wireless Communication Standards•IEEE 802.11 (Wi-Fi)•Bluetooth•Cellular networks (GSM, CDMA)Week 5: Building Wireless Communication Systems•Basic hardware components of a wireless communication system•Installing and configuring a wireless access point•Troubleshooting wireless network issues4. Teaching MethodologyThis course will be delivered through a combination of lectures, readings, assignments, and hands-on projects. Lectures will provide an introduction to the topic and cover the theoretical aspects of wireless communication. Readings will supplement the lectures and provide additional information on the course topics. Assignments will be given to evaluate the student’s understandin g of the course material. Hands-on projects will provide practical experience in building wireless communication systems.5. Assessment and GradingThe grade for this course will be based on the following components: Component WeightageAssignments 40%Hands-on Projects 30%Final Exam 30%6. References•Andreas F. Molisch,。
A Novel Drive Method for High-Speed Brushless DC Motor Operating in a Wide Range
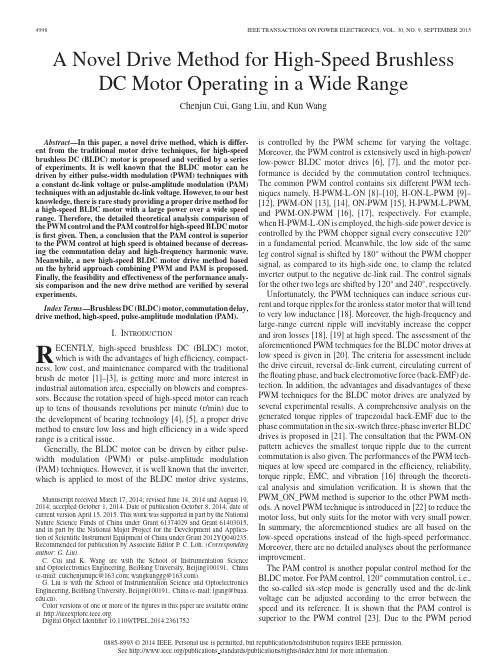
A Novel Drive Method for High-Speed BrushlessDC Motor Operating in a Wide RangeChenjun Cui,Gang Liu,and Kun WangAbstract—In this paper,a novel drive method,which is differ-ent from the traditional motor drive techniques,for high-speed brushless DC(BLDC)motor is proposed and verified by a series of experiments.It is well known that the BLDC motor can be driven by either pulse-width modulation(PWM)techniques with a constant dc-link voltage or pulse-amplitude modulation(PAM) techniques with an adjustable dc-link voltage.However,to our best knowledge,there is rare study providing a proper drive method for a high-speed BLDC motor with a large power over a wide speed range.Therefore,the detailed theoretical analysis comparison of the PWM control and the PAM control for high-speed BLDC motor isfirst given.Then,a conclusion that the PAM control is superior to the PWM control at high speed is obtained because of decreas-ing the commutation delay and high-frequency harmonic wave. Meanwhile,a new high-speed BLDC motor drive method based on the hybrid approach combining PWM and PAM is proposed. Finally,the feasibility and effectiveness of the performance analy-sis comparison and the new drive method are verified by several experiments.Index Terms—Brushless DC(BLDC)motor,commutation delay, drive method,high-speed,pulse-amplitude modulation(PAM).I.I NTRODUCTIONR ECENTLY,high-speed brushless DC(BLDC)motor, which is with the advantages of high efficiency,compact-ness,low cost,and maintenance compared with the traditional brush dc motor[1]–[3],is getting more and more interest in industrial automation area,especially on blowers and compres-sors.Because the rotation speed of high-speed motor can reach up to tens of thousands revolutions per minute(r/min)due to the development of bearing technology[4],[5],a proper drive method to ensure low loss and high efficiency in a wide speed range is a critical issue.Generally,the BLDC motor can be driven by either pulse-width modulation(PWM)or pulse-amplitude modulation (PAM)techniques.However,it is well known that the inverter, which is applied to most of the BLDC motor drive systems,Manuscript received March17,2014;revised June14,2014and August19, 2014;accepted October1,2014.Date of publication October8,2014;date of current version April15,2015.This work was supported in part by the National Nature Science Funds of China under Grant61374029and Grant61403015, and in part by the National Major Project for the Development and Applica-tion of Scientific Instrument Equipment of China under Grant2012YQ040235. Recommended for publication by Associate Editor P.C.Loh.(Corresponding author:G.Liu).C.Cui and K.Wang are with the School of Instrumentation Science and Optoelectronics Engineering,BeiHang University,Beijing100191,China (e-mail:cuichenjunupc@;wangkunggg@).G.Liu is with the School of Instrumentation Science and Optoelectronics Engineering,BeiHang University,Beijing100191,China(e-mail:lgang@buaa. ).Color versions of one or more of thefigures in this paper are available online at .Digital Object Identifier10.1109/TPEL.2014.2361752is controlled by the PWM scheme for varying the voltage. Moreover,the PWM control is extensively used in high-power/ low-power BLDC motor drives[6],[7],and the motor per-formance is decided by the commutation control techniques. The common PWM control contains six different PWM tech-niques namely,H-PWM-L-ON[8]–[10],H-ON-L-PWM[9]–[12],PWM-ON[13],[14],ON-PWM[15],H-PWM-L-PWM, and PWM-ON-PWM[16],[17],respectively.For example, when H-PWM-L-ON is employed,the high-side power device is controlled by the PWM chopper signal every consecutive120°in a fundamental period.Meanwhile,the low side of the same leg control signal is shifted by180°without the PWM chopper signal,as compared to its high-side one,to clamp the related inverter output to the negative dc-link rail.The control signals for the other two legs are shifted by120°and240°,respectively. Unfortunately,the PWM techniques can induce serious cur-rent and torque ripples for the ironless stator motor that will tend to very low inductance[18].Moreover,the high-frequency and large-range current ripple will inevitably increase the copper and iron losses[18],[19]at high speed.The assessment of the aforementioned PWM techniques for the BLDC motor drives at low speed is given in[20].The criteria for assessment include the drive circuit,reversal dc-link current,circulating current of thefloating phase,and back electromotive force(back-EMF)de-tection.In addition,the advantages and disadvantages of these PWM techniques for the BLDC motor drives are analyzed by several experimental results.A comprehensive analysis on the generated torque ripples of trapezoidal back-EMF due to the phase commutation in the six-switch three-phase inverter BLDC drives is proposed in[21].The consultation that the PWM-ON pattern achieves the smallest torque ripple due to the current commutation is also given.The performances of the PWM tech-niques at low speed are compared in the efficiency,reliability, torque ripple,EMC,and vibration[16]through the theoreti-cal analysis and simulation verification.It is shown that the PWM_ON_PWM method is superior to the other PWM meth-ods.A novel PWM technique is introduced in[22]to reduce the motor loss,but only suits for the motor with very small power. In summary,the aforementioned studies are all based on the low-speed operations instead of the high-speed performance. Moreover,there are no detailed analyses about the performance improvement.The PAM control is another popular control method for the BLDC motor.For PAM control,120°commutation control,i.e., the so-called six-step mode is generally used and the dc-link voltage can be adjusted according to the error between the speed and its reference.It is shown that the PAM control is superior to the PWM control[23].Due to the PWM period0885-8993©2014IEEE.Personal use is permitted,but republication/redistribution requires IEEE permission.See /publications standards/publications/rights/index.html for more information.interruption caused by the commutation and limitation of the res-olution of PWM generator,more torque ripple can be produced in the performance of PWM-controlled BLDC motor drives. This phenomenon will become more serious when the motors work at high speed.Moreover,it will lead to high motor loss. But it does not give enough theoretical analysis.The advantages and disadvantages of PWM and PAM are assessed in[24]using BLDC motor drives which are applied to the refrigerator.The result shows that the PAM control can provide higher efficiency than the PWM control.However,it only gives several low-power experimental results without any detailed analysis.A so-called PAM/PWM method[25]is proposed to control the adjustable speed drives.The realization of PAM relies on a current source rectifier which adjusts the dc-link voltage.As compared to the conventional PWM inverter with a constant dc-link voltage,this method can reduce the dc-link current ripples and prolong the lifetime of the capacitor effectively.However,the performance of controlling a BLDC motor is not involved.In order to improve the motor efficiency and provide the desired current waveform, a quasi-current source inverter is employed in[26]to adjust the dc-link voltage.The high-speed performance is improved,but the analysis and performance comparison with the PWM con-trol are not mentioned.A PWM-controlled buck-type dc-to-dc power converter in front of the three-phase bridge inverter is employed for the low-inductance and low-power BLDC mo-tor[27]–[29].The variable dc voltage is adjusted by the buck converter,and the inverter legs participate only in commutation instead of involved in modulation.But the detailed analysis is not discussed although the power consumption is reduced.To the best knowledge of the authors,the detailed analysis of whether the PWM control is suitable for the high-speed BLDC motor in a large speed range or not has not been fully inves-tigated.Therefore,it attracts the authors to give the analysis and performance comparison between the PWM control and the PAM control at high-speed region,thus providing a proper drive method in a wide speed range.In this paper,the theoretical analysis comparison between the PWM control and the PAM control at high-speed region isfirst given.Then,a new BLDC motor drive method over a wide speed range based on the analysis results is proposed.Therefore,the organization of this paper is as follows.Section II describes the detailed analysis about the advantages and disadvantages of the PWM control and the PAM control.Section III presents the new high-speed BLDC motor drive method.Section IV introduces the experimental results to validate the effectiveness and feasi-bility of the performance comparison analysis and the new drive method.Some important conclusions can be found in Section V.II.P ERFORMANCE A NALYSIS C OMPARISON B ETWEEN THE PWM C ONTROL AND THE PAM C ONTROLThe criteria of the performance comparison include the com-mutation delay analysis,harmonic component analysis,and mo-tor power factor analysis.mutation Delay AnalysisIn this paper,TI TMS320F28335digital signal processor (DSP)is taken as the controller to generate the PWMsignals.Fig.1.Scheme of the PWM generator based on the DSP.The frequency of PWM events is controlled by the time-base period(TBPRD)register and the mode of the time-base counter. The time-base counter has three modes of operation selected by the time-base control register,i.e.,up-down-count mode,up-count mode,and down-count mode.The up-down-count mode is selected to generate the triangu-lar carrier as shown in Fig.1.In this mode,the time-base counter starts from zero and increments until the period(TBPRD)value is reached.When the period value is reached,the time-base counter will decrement until it reaches zero.At this point, the counter repeats the pattern and begins to increment.When the time-base counter value is increasing to the counter-compare value,the output signal will be active high.On the contrary, when the time-base counter value is decreasing to the counter-compare value,the output signal will be active low.In the BLDC motor control system,if the PWM control is selected,the period value and the PWM period will be constant. The speed adjustment is achieved by changing the counter-compare value(counter-compare A/B register)to modulate the pulse width,i.e.,the duty ratio D.If a high-speed is needed,a large counter-compare value will be set to increase the output voltage.On the other hand,if a low speed is needed,a small counter-compare value will be set to decrease the output voltage. However,it is noted that if a new counter-compare value is written,the new value will not work immediately.Because the new value will befirst written to the shadow register associated with the counter-compare A/B register.Only when the counter value reaches zero,the new value will be loaded into the counter-compare A/B register from the shadow register.Assuming at a particular commutation process that the current transfers from phase C to phase A.This transfer is performed by switching OFF VT2and switching ON VT4.Before the transfer, VT3is ON and VT2is controlled by the chopper signal.When a commutation signal interruption occurs at t2as shown in Fig.1, theoretically,VT2should be turned OFF and VT4should be turned ON simultaneously.However,because the new counter value cannot work immediately until t4,the ideal conduction signal of VT4as shown in the dashed line of Fig.1cannot besatisfied.If the new counter-compare is loaded at t4,the counter starts from zero and increments until the new counter-compare value at t5when the actual conduction signal of VT4works and VT4is ON.Therefore,there is a lag time deviationΔt between the ideal conduction signal and the actual conduction signal, which can be expressed asΔt=t5−t2.(1) The lag angleδcaused byΔt can be expressed asδ=360T k·Δt(2)where T k is the electrical period of the motor.From(2),we can see that if the speed is low,δwill be very small and negligible due toΔt<T s<<T k(T s is the PWM period).However,if the speed is too high to ensure T s<T k,δwill be large enough becauseΔt may be close to the PWM period T k which is the same order of magnitude with T s.For example,for a motor with two pole pairs,if it runs at30000 r/min with a10k carrier frequency,T k will be1ms(T k=1/ 1000Hz)andδmay be close to36°.The largeδwill result in a large commutation delay.Even though this commutation delay can be neglected for a medium speed range,it has a significant influence on the phase current and drive performance at high speed,since the60°interval in which the commutation arises is relatively small.Moreover,the large lag angleδwill increase the motor loss and decrease the motor efficiency[29].In Fig.1,assuming that a commutation signal interruption occurs in the interval(t1,t4],we can also see that if the inter-ruption near t1,the largeΔt will lead to a largeδ,while if the interruption near t4,the smallΔt will lead to a smallδ.More-over,δis variable because the commutation signal interruption time varies with the speed.However,if the PAM control is employed,the PWM chopper signal will be no longer needed,sinceδcan be eliminated by set-ting the PWM generator port as the general-purpose input/output (I/O)port.When a commutation signal interruption occurs,the associated(I/O)port will immediately output a high/low level to turn ON/OFF the power device without delay.B.Harmonic Component AnalysisIn this paper,the adopted drive circuit structure for high-speed BLDC motor control is shown in Fig.2.For facilitate analysis,only the fundamental waves of the back-EMFs are considered,which can be expressed as⎧⎪⎨⎪⎩e Af=√2E sin(ωt)=E max sin(ωt)e Bf=√2E sin(ωt−2π/3)=E max sin(ωt−2π/3)e Cf=√2E sin(ωt+2π/3)=E max sin(ωt+2π/3)(3)where e Af,e Bf,and e Cf are the fundamental waves of the back-EMFs,E is the virtual value of the fundamental wave,E max=√2E is the maximum value of the fundamentalwave.Fig.2.Adopted drive circuit structure for high-speed BLDC motor control. u d is the output voltage of the rectifier,e A,e B,and e C are the back-EMFs, R is the phase-winding resistance,L is the phase-winding inductance,“N”is the neutral point,“S”is the virtual neutral point,“O”is the midpoint of the dc bus,and the voltage betwee n“S”and“O”is used to generated the sensorless commutation signals[30].Fig.3.Scheme of the PWM generator.Also,the fundamental waves of the line-to-line back-EMFs can be obtained as⎧⎪⎨⎪⎩e ABf=√6E sin(ωt+π/6)=√3E max sin(ωt+π/6)e BCf=√6E sin(ωt−π/2)=√3E max sin(ωt−π/2)e CAf=√6E sin(ωt+5π/6)=√3E max sin(ωt+5π/6)(4) where e ABf,e BCf,and e CAf are the fundamental waves of the line-to-line back-EMFs.For PWM control,the line-to-line voltages are modulated by the chopper signals.In this paper,u AB is taken as an example to be analyzed,and its fundamental wave can be expressed asu ABf=MU max sin(ωt+θ)(5)where u ABf is the fundamental wave of u AB,U max is the max-imum value of u ABf,0≤M≤1is the modulation ratio,andθis the angle between u ABf and e Af.The scheme of the PWM generator is shown in Fig.3,where α1andα2can be described as⎧⎪⎪⎨⎪⎪⎩α1=−π2−Mπsin(ωt+θ)2α2=π2+Mπsin(ωt+θ)2.(6)From Fig.3,u AO can be obtained by the Fourier decomposi-tion asu AO=u d2M sin(ωt+θ)+∞n=14nπ×sinMnπ2sin(ωt+θ)+nπ2cos(nωs t)(7)whereωs is the carrier angular frequency. Also,u BO can be obtained asu BO=u d2M sinωt−2π3+θ+∞n=14nπ×sinMnπ2sinωt−2π3+θ+nπ2cos(nωs t).(8)Therefore,u AB can be expressed asu AB=u AO−u BO=u ABf+u ABh(9) where u ABf and u ABh are the fundamental wave and the har-monic wave of u AB,respectively.From(7)–(9),u ABf and u ABh can be described asu ABf=u d2M sin(ωt+θ)−M sinωt−23π+θ=√32Mu d sinωt+θ+16π(10)u ABh=⎧⎪⎪⎪⎪⎪⎪⎪⎪⎪⎪⎪⎪⎪⎪⎪⎪⎪⎪⎨⎪⎪⎪⎪⎪⎪⎪⎪⎪⎪⎪⎪⎪⎪⎪⎪⎪⎪⎩u d2∞n=1(−1)(n+1)/24nπ∞k=2J kMnπ2×2sinkπ3sin(kω±nωs)t+kθ−π3(n=1,3,5,...,k=2,4,6,...,)u d2∞n=2(−1)n/24nπ∞k=1J kMnπ2×2sinkπ3cos(kω±nωs)t+kθ−π3(k=1,3,5,...,n=2,4,6,...,)(11)where J k is the k-order Bessel function.From(11),we can see that the harmonic waves with the frequency of kω±nωs are introduced because of the PWM control.The equivalent circuit of the fundamental wave and the har-monic wave are shown in Fig.4(a)and(b),respectively.It can be seen that the distorted voltage u ABh will induce the distorted current i ABh,which will inevitably increase the copper loss of the motor.It is well known that the iron loss of the motor also increases with the frequency.Therefore,the harmonic wave will increase both the copper loss and the iron loss.C.Motor Power Factor AnalysisThe vector diagram illustrating the relationship between u AB and i AB(i AB=i A)after the transfer is shown in Fig.5.Fig.4.Equivalent circuit of the fundamental wave and the harmonic wave.(a)Fundamental wave equivalent circuit.(b)Harmonic wave equivalentcircuit. Fig.5.Vector diagram about the relationship between u A B and i A B after thetransfer.Generally,the power factor of the fundamental component, i.e.,the displacement factor,can be obtained from the angle between U AB and I AB as shown in Fig.5and expressed asγ=cosψ1(12) whereγis the displacement factor andψ1is the displacement factor angle.However,if the PWM control is employed,the actual current I AB will lag the ideal current I AB of aboutδelectrical degrees due to the lag angle mentioned above.Therefore,the actual displacement factor angle equalsψ1+δ,and the displacement factor can be expressed asγ=cos(ψ1+δ).(13) It is obvious that the PWM control decreases the displacement factor.In addition,we should note that(12)and(13)are only asso-ciated with the fundamental component.If the harmonics wave is considered,the motor power factor can be expressed as PF=PS=U1I1cosΦ1+nh=2U h I h cosΦhUI(14) where PF is the power factor,P is the active power,S is the ap-parent power,andΦ1=ψ1+δis the displacement factor angle. U is the RMS magnitude of the line-to-line voltage and U1is the RMS magnitude of the fundamental component,I is the RMS magnitude of the total current and I1is the RMS magnitude of the fundamental component,U h is the RMS magnitude of the line-to-line voltage harmonic component and I h is the RMS magnitude of the current harmonic component,andΦh is the angle between U h and I h.TABLE IM OTOR P ARAMETERSSurface Mounted BLDC MotorRated DC voltage480VRated current218ARated speed,n32000r/minNumber of pole pairs,P2Phase resistance,R 1.5mΩPhase inductance,L0.053mHLine-to-line back-EMF constant,K e-l i n e0.0127V/r/minMoment of inertia,J0.024059kg·m2mon drive circuit structure for the BLDC motor control.Thefirst term,i.e.,U1I1cosΦ1,shows the main power term, which is associated with the fundamental frequency.The second (summation)term accounts for the harmonics powers absorbed by the loads and other system components.Because of the PWM control,the lag angleδand the harmonic component decrease the power factor.It is well known that when the active power is constant,the small power factor will increase the reactive power,apparent power,and motor loss.III.P ROPOSED D RIVE M ETHODA.Drive Circuit Selection of High-Speed BLDC MotorAs analyzed above,the PAM control for the BLDC motor can provide less motor loss and higher efficiency than the PWM control at high speed.So the PAM control should be employed, especially at high speed.However,from the specification of the experimental BLDC motor as shown in Table I,it is interesting tofind that the phase-winding resistance and the phase-winding inductance of high-speed BLDC motor are far smaller than those of the common low-speed BLDC motor.When the motor starts, the initial DC voltage of the three-phase inverter should be smaller enough to limit the startup current,as the back-EMF is too small to counteract a large dc voltage at the very low speed.Therefore,a suitable drive circuit should befirst selected to reduce the initial dc voltage and guarantee the safe motor starting process.During the experimental process,the authors have tried three drive circuit structures to limit the startup current as shown in Figs.2,6,and7.Fig.6shows the common drive circuit structure for the BLDC motor control.The dc voltage,i.e.,the output voltage of the three-phase uncontrolled rectifier,is more than500V.Though the three-phase inverter is controlled by the PWM scheme for reducing the voltage,the startup current is still far larger thanthe Fig.7.Drive circuit structure based on the dc–dc converter for the BLDC motor control.rated current.Moreover,the inverter cannot work anymore,as the protection circuit blocks the inverter due to the large current. So this common circuit structure is not employed.Fig.7shows the drive circuit structure based on a front-end PWM-controlled dc–dc converter.A buck converter is taken to reduce the dc voltage of the three-phase inverter.Moreover, the motor starts normally.Unfortunately,the buck converter in-creases the complexity mainly due to the dc inductance,L B,as marked in Fig.7.Let us assume that the working frequency of the buck converter is6kHz.As the rated power of the exper-imental motor is up to100kW,the normal dc currentflowing through L B may be more than250A which will cause the bulky size and weight as shown in Fig.7.Moreover,the bulky L B will increase the difficulty of the whole circuit layout design because the unreasonable layout will induce strong electromagnetic in-terference,which may affect the normal operation of high-speed and high-power BLDC motor.Finally,taking into account the subsequent PAM control and the disadvantages of the above-mentioned circuit structures,a motor drive circuit as shown in Fig.2is employed in this pa-per.The front-end phase-controlled rectifier can provide an ad-justable dc-link voltage to limit the startup current and achieve the PAM control,while the back-end three-phase inverter can achieve the commutation control.B.Proposed Drive MethodIn order to satisfy the commutation control of high-speed BLDC motor in a wide speed range and improve the motor ef-ficiency,a hybrid drive method combining PWM and PAM is proposed.When the motor runs at low speed,the PWM control is adopted with afixed dc-link voltage.With the motor accel-erating,the back-EMF will increase with the speed.When the motor speed reaches a threshold value,i.e.,the enough back-EMF can counteract the dc-link voltage,the PAM control works with an adjustable dc-link voltage.It should be noted that though the PAM control for the BLDC motor is superior to the PWM control,the PAM control is not employed in the whole speed range for the following two reasons.Thefirst one is that the larger dc-link voltagefluctuation of the rectifier limits its application at low and medium speed. Moreover,at low speed,it may be unable to produce a small enough dc voltage to achieve the PAM control due to the smallFig.8.Hysteresis loop schematic diagram.phase-winding resistance and phase-winding inductance.There-fore,if we want to take the PAM control in a wide speed range, the range of dc-link voltage should be expanded at medium and low speed.Meanwhile,a front-end PWM-controlled dc–dc con-verter as shown in Fig.7is needed to produce such a low dc voltage.But the complexity of the system design is increased as explained above.The second one is that,to our best knowledge,the PWM control is the auxiliary drive method of high-speed BLDC motor, while the PAM control is the main drive method,since the high-speed region is the most common working range.The aim of the PWM control is merely to guarantee that the high-speed motor can be accelerated to a high speed.Therefore,the operation time of the PWM control is far shorter than that of the PAM control, thus mitigating the disadvantage of the PWM control.However, both the two drive methods are indispensable.The transition process between PWM and PAM is achieved by a hysteresis comparator expressed asτ(n(k+1))=⎧⎪⎨⎪⎩1,(n(k+1)>n+)τ(n(k)),(n−≤n(k+1)≤n+)0,(n(k+1)<n−)(15)whereτ(n(k+1))is the(k+1)th hysteresis comparator out-put value,τ(n(k))is the(k)th hysteresis comparator output value, n−and n+are the two endpoint speeds of the hysteresis com-parator,n(k+1)is the(k+1)th calculated speed value,and n(k)is the(k)th calculated speed value.The hysteresis loop schematic diagram is shown in Fig.8.The region between n−and n+is defined as the transition region. Ifτ(n(k+1))=1,the PAM control mode will start to work, while ifτ(n(k+1))=0,the PWM control mode will start to work.C.Calculation Principle for n+From Fig.2,we can obtain⎧⎪⎨⎪⎩u AN=Ri A+Ldi A/dt+e Au BN=Ri B+Ldi B/dt+e Bu CN=Ri C+Ldi C/dt+e C(16)where u AN,u BN,and u CN are the three phase voltages.Let us assume that VT1and VT6are switched ON,i.e., phases A and B are conducted simultaneously with i A=−i B and i C=0.So the equivalent circuit can be shown in Fig.9.Fig.9.Equivalent circuit when VT1and VT6are switchedON.Fig.10.Experimental platform.(a)Control system.(b)Experimental motors.From Fig.9,the line-to-line voltage can be obtained asu d(0)=u AB=2Ri A+2Ldi A/dt+e ABe AB=k e-line n+(17)where u d(0)is the initial voltage of the rectifier,i.e.,thefixed dc-link voltage when the PWM control works,and k e-line is the line-to-line back-EMF constant.Therefore,we can obtain the online result of n+by solving (17).In this paper,n+is about10000r/min.Meanwhile,n−can be obtained as n−=n+−Δn(Δn is the hysteresis width).D.Calculation Principle forΔnThe motor torque balance equation of the BLDC motor can be obtained asT M−T Z=JdΩdt=2π60Jdndt(18) where T M is the electromagnetic torque,T Z is the load torque, J is the moment of inertia,andΩis the mechanical angular velocity of the motor.Generally,for a BLDC motor,T M can be expressed asT M=(e A i A+e B i B+e C i C)/Ω=60(e A i A+e B i B+e C i C)/(2πn).(19) Considering(17),i A=−i B,and i C=0,we can also obtain T M asT M=60i A(u AB−2Ri A−Ldi A/dt)/(2πn).(20) It can be deduced from[29]that the commutation delay in-duced by the PWM control will lead to a serious torque ripple. So when the drive method transfers from the PWM control to the PAM control,a torque ripple,ΔT M,will be introduced,and (18)can be expressed asT M+ΔT M−T Z=JdΩdt=2π60Jdndt.(21)Fig.11.Waveform of i A and u A B at different speeds.(a)PWM n=6000r/min.(b)PWM n=24000r/min.(c)PAM n=24000r/min. From(21),we canfind that ifΔT M>0,the speed willincrease,while ifΔT M<0,the speed will decrease.Unfortu-nately,ΔT M is uncertain during the transition.It can be seenfrom Fig.8that when the speed reaches n+,if the speed is decel-erating,the drive method may switch between the PAM controland the PWM control repetitiously which aggravates the cur-rent ripple.Therefore,a proper hysteresis width is critical toeliminate the unexpected repetitious switching.The proper hysteresis width is selected from many initial ex-periments,of which accelerate and decelerate the motor to passthe transition region with different hysteresis widths automat-ically,when the motorfirst runs.OnceΔn is selected,it willbe saved and does not need to be calculated in the subsequentexperiments.Considering(21),Δn can be obtained asΔn=λΔt·dndt+λ1Δn1=λΔt·602πJ×(T M+ΔT M−T Z)+λ1Δn1(22) whereλ=1.5−2is the experiential safety factor of the speed fluctuation during the transition,Δt=T s/6is the commutation interval,T M is the electromagnetic torque that can be obtained from(20),ΔT M=−(1−2)T Z is a experiential worst torque ripple that the motor can endure,λ1=1.2−1.5is the experi-ential rate accuracy safety factor,andΔn1=(1−3%)n+is the rate accuracy.In this paper,Δn is set500r/min according to(22).IV.E XPERIMENTAL E V ALUATIONA.Experiment SetupThe experiment about the performance analysis comparison and the proposed drive method has been successfully imple-mented on the experimental high-speed magnetically suspended BLDC motor that coupled to a magnetically suspended gener-ator by aflexible coupling.A variable resistance is taken as the load.The specification of the experimental BLDC motor is shown in Table I,while the experimental platform is shown in mutation Delay ValidationFig.11(a)shows the waveform of i A and u AB at6000r/min under PWM control with the dc voltage equal to450V,while Fig.11(b)shows that at24000r/min under PWM control.As explained above,the PWM control will lead to a commutation delay.Moreover,although this commutation delay can be ne-glected in a medium speed range,it has a significant influence on the phase current and the drive performance at high speed. When the speed is low as shown in Fig.11(a),the commuta-tion delay is unconspicuous.However,when the speed is high as shown in Fig.11(b),the commutation delay is so obvious that i A and u AB are seriously distorted.It is well in accord with the operation given in[31]–[33]when the lagged commu-tation occurs.Fig.11(c)shows the operation at24000r/min with PAM control.It can be seen that the commutation delay is eliminated and the distortion of i A and u AB is improved greatly.C.Harmonic Content ValidationFig.12shows the spectrum map comparison of i A and u AB between the PAM control and the PWM control at different speeds.Fig.12(a)and(b)shows the spectrums at12000r/min. We can see that the harmonic component under PWM control is larger than that under PAM control.The THD of i A decreases from1.245to0.663,while that of u AB decreases from1.955 to0.679.Meanwhile,Fig.12(c)and(d)shows the spectrums at20000r/min.Also,the harmonic component under PWM control is serious.We can alsofind from these fourfigures that when the PWM control is employed,the THD will decreases at high speed.To our best knowledge,this is because that the duty ratio can be maintained at higher level at high speed with the same generator load resistor.However,the improvement of THD is more insignificant than that under PAM control.Fig.12(e) and(f)shows the spectrums at24000r/pared with the conditions at12000and20000r/min,the load is heavy due to the high speed.Nevertheless,the PAM control can still decrease the harmonic content greatly.In addition,it is noted that the THD under PAM control at20000r/min is lower than that at24000r/min.Therefore,for PAM control,the THD is not decreased with speed.The relationship between the THD and the speed under PAM control needs further research.。
外文翻译数字频率合成器

附录2:外文原文,译文Modulating Direct Digital Synthesizer In the pursuit of more complex phase continuous modulation techniques, the control of the output waveform becomes increasingly more difficult with analog circuitry. In these designs, using a non-linear digital design eliminates the need for circuit board adjustments over yield and temperature. A digital design that meets these goals is a Direct Digital Synthesizer DDS. A DDS system simply takes a constant reference clock input and divides it down a to a specified output frequency digitally quantized or sampled at the reference clock frequency. This form of frequency control makes DDS systems ideal for systems that require precise frequency sweeps such as radar chirps or fast frequency hoppers. With control of the frequency output derived from the digital input word, DDS systems can be used as a PLL allowing precise frequency changes phase continuously. As will be shown, DDS systems can also be designed to control the phase of the output carrier using a digital phase word input. With digital control over the carrier phase, a high spectral density phase modulated carrier can easily be generated.This article is intended to give the reader a basic understanding of a DDS design, and an understanding of the spurious output response. This article will also present a sample design running at 45MHz in a high speed field programmable gate array from QuickLogic.A basic DDS system consists of a numerically controlled oscillator (NCO) used to generate the output carrier wave, and a digital to analog converter (DAC) used to take the digital sinusoidal word from the NCO and generate a sampled analog carrier. Since the DAC output is sampled at the reference clock frequency, a wave form smoothing low pass filter is typically used to eliminate alias components. Figure 1 is a basic block diagram of a typical DDS system generation of the output carrier from the reference sample clock input is performed by the NCO. The basic components of the NCO are a phase accumulator and a sinusoidal ROM lookup table. An optional phase modulator can also be include in the NCO design. This phase modulator will add phase offset to the output of the phase accumulator just before the ROM lookup table. This will enhance the DDS system design by adding the capabilities to phase modulate the carrier output of the NCO. Figure 2 is a detailed block diagram of a typical NCO design showing the optional phase modulator.FIGURE 1: Typical DDS System.FIGURE 2: Typical NCO Design.To better understand the functions of the NCO design, first consider the basic NCO design which includes only a phase accumulator and a sinusoidal ROM lookup table. The function of these two blocks of the NCO design are best understood when compared to the graphical representation of Euler’s formula ej wt = cos( wt) + jsin( wt). T hegraphical representation of Euler’s formula, as shown in Figure 3, is a unit vector rotating around the center axis of the real and imaginary plane at a velocity of wrad/s. Plotting the imaginary component versus time projects a sine wave while plotting the real component versus time projects a cosine wave. The phase accumulator of the NCO is analogous, or could be considered, the generator of the angular velocity component wrad/s. The phase accumulator is loaded, synchronous to the reference sample clock, with an N bit frequency word.This frequency word is continuously accumulated with the last sampled phase value by an N bit adder. The output of the adder is sampled at the reference sample clock by an N bit register. When the accumulator reaches the N bit maximum value, the accumulator rolls over and continues. Plotting the sampled accumulator values versus time produces a saw tooth wave form as shown below in Figure 3. FIGURE 3 Euler’s Equation Represented GraphicallyThe sampled output of the phase accumulator is then used to address a ROM lookup table of sinusoidal magnitude values. This conversion of the sampled phase to a sinusoidal magnitude is analogous to the projection of the real or imaginary component in time. Since the number of bits used by the phase accumulator determines the granularity of the frequency adjustment steps, a typical phase accumulator size is 24 to 32 bits. Since the size of the sinusoidal ROMtable is directly proportional to the addressing range, not all 24 or 32 bits of the phase accumulator are used to address the ROM sinusoidal table. Only the upper Y bits of the phase accumulator are used to address the sinusoidal ROM table, where Y < N bits and Y is typically but not necessarily equal to D, and D is the number of output magnitude bits from the sinusoidal ROM table.Since an NCO outputs a carrier based on a digital representation of the phase and magnitude of the sinusoidal wave form, designers have complete control over frequency, phase, and even amplitude of the output carrier. By adding a phase port and a phase adder to the basic NCO design, the output carrier of the NCO can be M array phase modulated where M equals the number of phase port bits and where M is less than or equal to the Y number of bits used to address the sinusoidal ROM table. For system designs that require amplitude modulation such as QAM, a magnitude port can be added to adjust the sinusoidal ROM table output. Note that this port is not shown in Figure 2 and that this feature is not demonstrated in the sample QuickLogic FPGA design. Finally, frequency modulation is a given with the basic NCO design. The frequency port can directly adjust the carrier output frequency. Since frequency words are loaded into the DDS synchronous to the sample clock, frequency changes are phase continuous.Although DDS systems give the designer complete control ofcomplex modulation synthesis, the representation of sinusoidal phase and magnitude in a non-linear digital format introduces new design complexities. In sampling any continuous-time signal, one must consider the sampling theory and quantization error.To understand the effects of the sampling theory on a DDS system, it is best to look at the DDS synthesis processes in both the time and frequency domain. As stated above, the NCO generates a sinusoidal wave form by accumulating the phase at a specified rate and then uses the phase value to address a ROM table of sinusoidal amplitude values. Thus, the NCO is essentially taking a sinusoidal wave form and sampling it with the rising or falling edge of the NCO input reference sampling clock. Figure 4 shows the time and frequency domain of the NCO processing. Note that this representation does not assume quantization.Based on the loaded frequency word, the NCO produces a set of amplitude output values at a set period. The frequency domain representation of this sinusoid is an impulse function at the specified frequency. The NCO, however, outputs discrete digital samples of this sinusoid at the NCO reference clock rate. In the time domain, the NCO output is a function of the sampling clock edge strobes multiplied by the sinusoid wave form producing a train of impulses at the sinusoid amplitude. In the frequency domain, the sampling strobes of the reference clock produce a train of impulses at frequencies of K times theNCO clock frequency where K = ... - 1, 0, 1, 2 .... Since the sampling clock was multiplied by the sinusoid in the time domain, the frequency domain components of the sinusoid and the sampling clock need to be convolved to produce the frequency domain representation of the NCO output.The frequency domain results are the impulse function at the fundamental frequency of the sinusoid and the alias impulse functions occurring at K times the NCO clock frequency plus or minus the fundamental frequency. The fundamental and alias component occur at: K*Fclk - FoutK*Fclk + FoutWhere K = ... -1, 0 , 1, 2 ..... and K = 0 is the NCO sinusoid fundamental frequencyFout is the specified NCO sinusoid output frequencyFclk is the NCO reference clock frequencyFIGURE 4 NCO Output Representation Time and Frequency Domain The DAC of the DDS system takes the NCO output values and translates these values into analog voltages. Figure 4 shows the time and frequency domain representations of the DAC processing starting with the NCO output. The DAC output is a sample and hold circuit that takes the NCO digital amplitude words and converts the value into an analog voltage and holds the value for one sample clock period. The timedomain plot of the DAC processing is the convolution of the NCO sampled output values with a pulse of one sample clock period. The frequency domain plot of the sampling pulse is a sin(x)/x function with the first null at the sample clock frequency. Since the time domain was convolved, the frequency domain is multiplied. This multiplication dampens the NCO output with the sin(x)/x envelope. This attenuation at the DAC output can be calculated as follows and a sample output spectrum is shown in Figure 5:Atten(F) = 20log[(sin(pF/Fclk)/pF/Fclk)] Where F is the output frequency Fclk is the sample clock frequencyFIGURE 5: DAC Output Representation in Time and Frequency Domain Aside from the sampling theory, the quantization of the real values into digital form must also be considered in the performance analysis of a DDS system. The spurious response of a DDS system is primarily dictated by two quantization parameters. These parameters are the phase quantization by the phase accumulator and the magnitude quantization by the ROM sinusoidal table and the DAC.As mentioned above, only the upper Y bits of the phase accumulator are used to address the ROM lookup table. It should be noted, however, that using only the upper Y bits of the phase accumulator introduces a phase truncation. When a frequency word containing a non-zero value in the lower (N-Y-1:0) bits is loaded into the DDS system, the lowernon-zero bits will accumulate to the upper Y bits and cause a phase truncation. The frequency at which the phase truncation occurs can be calculated by the following:Ftrunc = FW(N-Y- 1:0)/2N-Y* Fclk.A phase truncation will periodically (at the Ftrunc rate) phase modulate the output carrier forward 2p/28 to compensate for frequency word granularity greater than 2Y. The phase jump caused by the accumulation of phase truncated bits produces spurs around the fundamental.These spurs are located plus and minus the truncation frequency from the fundamental frequency and the magnitude of the spurs will be - 20log(2Y)dBc. A sample output of a phase truncation spur is shown in Figure 5.In a typical NCO design, the ROM sinusoidal table will hold a ¼ sine wave (0 , p/2) of magnitude values. The ROM table is generated by taking all possible phase value addresses and map to a real magnitude sine value rounded to the nearest D bits. Thus, the maximum error output is ±- ½ LSB giving a worst case spur of -20log(2D)dBc.Like the NCO ROM table, a DAC quantizes the digital magnitude values. A DAC, however, outputs an analog voltage corresponding to the digital input value. When designing the NCO sinusoidal ROM table, one should take some empirical data on the DAC linearity to betterunderstand the interaction between the ROM table and the DAC. The quantization for a DAC is specified against an ideal linear plot of digital input versus analog output. Two linearity parameters, differential and integral linearity, are used to specify a DAC’s p erformance.Differential linearity is the output step size from bit to bit. A DAC must guarantee a differential linearity of a maximum 1 LSB. When an input code is increased, the DAC output must increase. If the DAC voltage does not increase versus an increase digital input value, the DAC is said to be missing codes. Thus, a 10 bit DAC that has a differential linearity of greater that 1 LSB is only accurate to 9 or less bits. The number of accurate output bits will specify the DDS spurious performance as -20log(2dl) where dl is the number differential linear bits..Integral linearity is a measure of the DAC’s overall linear performance versus an ideal linear straight line. The straight line plot can be either a “best straight line” where DC offsets are pos sible at both the min and max outputs of the DAC, or the straight line can cross the end points of the min and max output values. A DAC will tend to have a characteristic curve that is traversed over the output range. Depending on the shape and symmetry (symmetry about the half way point of the DAC output) of this curve, output harmonics of the DDS fundamental output frequency will be produced. As these harmonics approach andcross the Nyquist frequency of Fclk/2, the harmonics become under sampled and reflect back into the band of interest, 0 to Fclk/2. This problem is best illustrated by setting the NCO output to Fclk/4 plus a slight offset. The third harmonic will fall minus 3 folds the small offset from the fundamental and the second harmonic will cross the Nyquist frequency by 2 folds the small offset leaving a reflected image back in the band of interest A sample plot of this frequency setup is shown in Figure 5.Other DAC characteristic that will produce harmonic distortion is any disruption of the symmetry of the output wave form such as a different rise and fall time. These characteristics can typically be corrected by board components external to the DAC such as an RF transformer, board layout issues, attenuation pads etc.Given the complexities of the DDS system, engineers should consider implementing the design using separate devices for the numerically controlled oscillator, the digital to analog converter, and the low pass filter. This approach allows for signal observation at many points in the system, yet is compact enough to be practical as an end-solution. Alternatively, the discrete implementation can serve as a prototyping vehicle for a single-chip mixed signal ASIC.The author developed a version of the design using a Harris HI5721 evaluation board for the DAC. The NCO at the heart of the DDS design,and a random generator to test signal modulation, was implemented into about 65% of a QuickLogic field programmable gate array (FPGA). This FPGA, a QL16x24B 4000-gate device, was chosen for its high performance, ease-of-use, and powerful development tools.The NCO design included following:Developed in Verilog with the 8 bit CLA adder schematiccaptured and net listed to Verilog32 bit frequency word input32 phase accumulator pipelined over 8 bits8 bit phase moudulation word input8 bit sine ROM look-up tableThe design was described mostly in Verilog, with an 8 bit carry look ahead adder modified from QuickLogic’s macro library netlisted to Verilog. The whole design cycle was less than four days (two days to describe the design and a day and a half to prototype the hardware). Everything worked perfectly the first time, with the design running at an impressive 45MHz as predicted by the software simulation tools.Plots used in the article to illustrate DDS performance parameters were provided from the test configuration.Figure 6 below shows the external IO interface to the NCO design .The function of each signal is described in the following table.Signal Function TableFigure 6: The External IO InterfaceTop LevelThe top level of the NCO design instantiates the functional blocks of the NCO design and the PN generator block.PN GeneratorThis module is not part of the NCO design but is used to produce a sample random data pattern to modulate the carrier output. This module uses the PNCLK input to clock two Gold code 5 bit PN generators. The outputs of the PN generators are IDATA and QDATA outputs.The lower level block of this NCO design consist of a synchronous frequency word input register, a synchronous phase word input register, a 32 bit pipe lined phase accumulator, an 8 bit phase adder, and a sin lockup table. A detailed description of each of the NCO blocks and thePN generator are provided in the following sections.Load Frequency WordThe load frequency word block is a synchronizing loading circuit. The FREQWORD[31:0] input drives a the data input to the 32 bit fwreg register that is sampled on the rising edge of the FWWRN write strobe. The FWWRN strobe also drives the data input to a metastable flip flop fwwrnm that is used in conjunction with a synchronous register fwwrns to produce a FWWRN rising edge strobe. This rising edge strobe loadp1 is then piped for an additional 3 clock cycles producing the load strobes loadp2, loadp3, and loadp4. The load strobes are used to signal when to update the synchronous pipe line 8 bit registers pipefw1, pipefw2, pipefw3, and pipefw4 to the sampled frequency word content. The pipe line registers are concatenated to produce the 32 bit synchronous frequency word output SYNCFREQ[31:0] that is staggered to compensate for the 32 bit pipe lined phase adder.Phase Word AccumulatorThe phase accumulator block is a 32 bit accumulator that is pipe lined in 8 bit sections. This module instanciates a schematic captured carry lock ahead CLA adder that has a carry in and carry out port. The synchronous frequency word, staggered to match the pipe lined accumulator, is loaded into the B input of the CLA adders. The sum output of the CLA adders are registered in the pipe registered with theoutput tied back to the A input of the CLA adders. The carry output of the CLA adders is registered in the pipec registers with the output tied to the next most significant CLA adder carry input. The most significant sum output register pipe4 is assigned to the PHASE output port giving a phase value quantized to 8 bits. A digital sine and cosine value is also calculated from the pipe4 register and brought out of the chip as SIN and COS.Load Phase WordThe load phase word block is a synchronizing loading circuit. The PHASEWORD[7:0] input drives the data input to the 32 bit pwreg register that is sampled on the rising edge of the PWWRN write strobe. The PWWRN strobe also drives the data input to a metastable flip flop pwwrnm that is used in conjunction with a synchronous register pwwrns to produce a FWWRN rising edge strobe. This rising edge strobe load is used to signal when to update the synchronous phase word register phswd. The phswd register is assigned to the synchronous phase word output SYNCPHSWD[7:0].Phase ModulatorThe phase modulator block is used to phase offset the phase accumulator 8 bit quantized output with the synchronous phase word from the load phase word block. This module instantiates a CLA adder with the A input tied to the synchronous phase output and the B inputtied to the phase accumulator output. The sum output of the adder is registered in the mphsreg register and assigned to the MODPHASE output port. A modulated version of the sine and cosine values are calculated and brought out of the chip as MSIN and MCOS.Sine LockupThis module takes the modulated phase value form the phase modulator block and translated the quantized 8 bit value into a sine wave form amplitude value quantized to 8 bits. The translation from phase to amplitude is performed by a sine ROM table that in instantiated in this module. The ROM table is reduced to a ¼ of the symmetrical sine wave form and the MSB of the sine wave form is equivalent to the modulated phase module performs the calculations to reconstruct a complete period of the sine wave form f rom the ¼ representation of the ROM table and the MSB of the modulated phase input. To better understand the processing of this module, consider the following. The modulated phase value is a 0 to 2p value quantized to 8 bits 2p/28. The quantized value for p/2, p, 3p/2, and 2p are 0x3F, 0x7F, 0xBF, and 0xFF. The amplitude values for 0 to p/2 is stored in the ROM table. The amplitude values for p/2 to p are the ROM table output in the reverse order. The amplitude values for p to 3p/2 are the same output as the amplitude value from 0 to p/2 with the output from the ROM table inverted. Finally the amplitude value for 3p/2 to 2p are the same as for pto 3p/2 with the ROM table accessed in reverse.This module manages the address values to the ROM table and the amplitude outputs to form the complete period of the sine wave form. The first process of generating the sine wave function is the addressing of the ROM table such that phase angles p/2 to p and 3p/2 to 2p are addressed in the reverse order. Reverse addressing is accomplished by simply inverting the ROM table address input vector. The phase modulated address input is inverted when the MODPHASE[6] is one and is then registered in the phaseadd register. The phase address is used to address the ROM sine table with the output registered in the qwavesin_ff register. To construct the negative amplitude values of the sine wave form, the MSB of the modulate phase word input is registered twice in modphase_msb1_ff and modphase_msb2_ff, compensating for the two cycle latency of the phaseadd and qwavesin_ff registers. The delayed MSB bit is used to invert the ROM table output when one. The altered ROM table output and the invert of the delayed modulated phase word MSB are finally registered in by the dac_ff register and then assigned to the DACOUT output port.Sine ROM TableThis module is the sine wave form ROM table. This table converts the phase word input to a sine amplitude output. To conserve area, only ¼ of the symmetrical sine wave form is stored in the ROM. The sine valuesstored in this table are the 0 to p/2 unsigned values quantized to 8 bits. Thus, the ROM table requires a 6 bit phase address input and outputs a 7 bit amplitude output. The sinlup module processes the phase and amplitude values to produce a complete sine period.Dan Morelli has over 9 years of design and management experience. His areas of expertise include spread spectrum communications (involving GPS, TDRSS, and , PC chip set and system architecture, cell library development (for ECL devices) and ASIC development. He has been published and has multiple patents awarded and pending. Dan currently works for Accelent Systems Inc., an electronic design consulting company, where he is a founder and the VP of Engineering.数字频率合成器在探讨许多复杂的相位持续的调制技术中,对模拟电路中输出波形的操纵已经愈来愈困难。
通信专业英语-Lesson 6
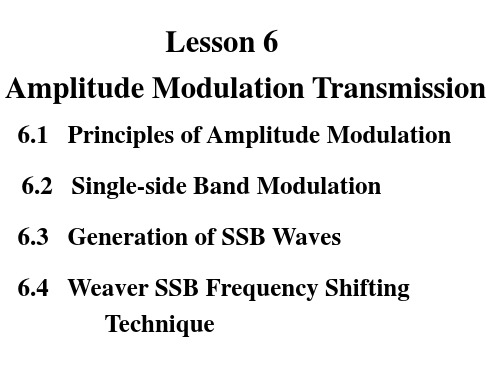
The bandwidth (B) of an AM DSBFC wave is equal to the difference between the highest upper side frequency and the lowest side frequency, or two times the highest modulating signal frequency .
Technique
Introduction
Q1: What is the purpose of modulation? Q2: What is modulation? Q3: What is demodulation? Q4: What is AM? Q5: What applications can the AM be used for? Q6: What is the construction of the AM modulator? Q7: What’s the definition of RF?
Lesson 6 Amplitude Modulation Transmission
6.1 Principles of Amplitude Modulation 6.2 Single-side Band Modulation 6.3 Generation of SSB Waves 6.4 Weaver SSB Frequency Shifting
直译与意译
This means that insofar as the transmission of information is concerned, only one sideband is necessary, and if the carrier and the other sidebands are suppressed at the transmitter, no information is lost.
科技英语的翻译——01 科技英语的语法特点
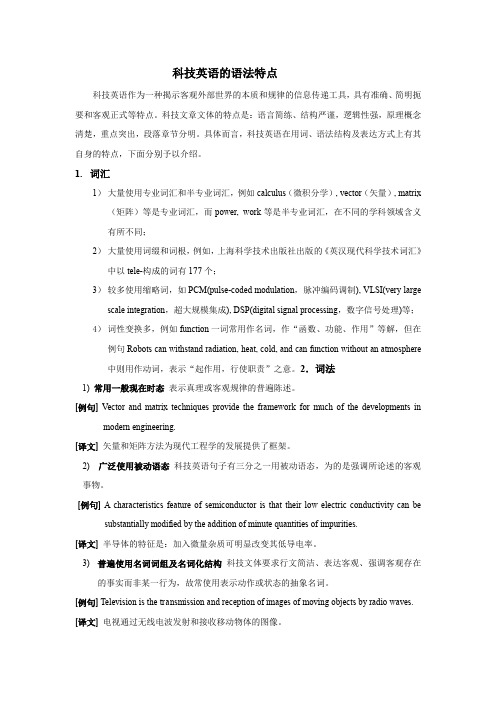
科技英语的语法特点科技英语作为一种揭示客观外部世界的本质和规律的信息传递工具,具有准确、简明扼要和客观正式等特点。
科技文章文体的特点是:语言简练、结构严谨,逻辑性强,原理概念清楚,重点突出,段落章节分明。
具体而言,科技英语在用词、语法结构及表达方式上有其自身的特点,下面分别予以介绍。
1.词汇1)大量使用专业词汇和半专业词汇,例如calculus(微积分学), vector(矢量), matrix (矩阵)等是专业词汇,而power, work等是半专业词汇,在不同的学科领域含义有所不同;2)大量使用词缀和词根,例如,上海科学技术出版社出版的《英汉现代科学技术词汇》中以tele-构成的词有177个;3)较多使用缩略词,如PCM(pulse-coded modulation,脉冲编码调制), VLSI(very large scale integration,超大规模集成), DSP(digital signal processing,数字信号处理)等;4)词性变换多,例如function一词常用作名词,作“函数、功能、作用”等解,但在例句Robots can withstand radiation, heat, cold, and can function without an atmosphere中则用作动词,表示“起作用,行使职责”之意。
2.词法1) 常用一般现在时态表示真理或客观规律的普遍陈述。
[例句]Vector and matrix techniques provide the framework for much of the developments in modern engineering.[译文]矢量和矩阵方法为现代工程学的发展提供了框架。
2) 广泛使用被动语态科技英语句子有三分之一用被动语态,为的是强调所论述的客观事物。
[例句] A characteristics feature of semiconductor is that their low electric conductivity can be substantially modified by the addition of minute quantities of impurities.[译文] 半导体的特征是:加入微量杂质可明显改变其低导电率。
模块化多电平换流器(MMC)调制方法综述
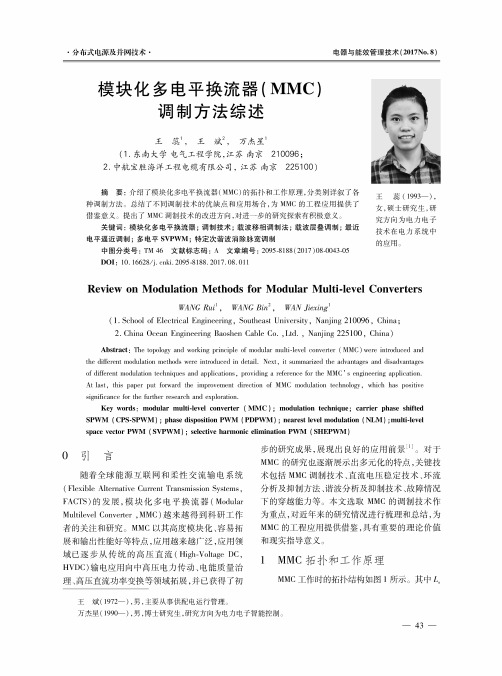
•分布式电源及并网技术!电器与能效管理技术(2017%). 8)模块化多电平换流器(MMC )调制方法综述王蕊1,王斌2,万杰星1(!东南大学电气工程学院,江苏南京210096;2.中航宝胜海洋工程电缆有限公司,江苏南京225100)摘要:介绍了模块化多电平换流器(MMC )的拓扑和工作原理,分类别详叙了各种调制方法。
总结了不同调制技术的优缺点和应用场合,为MMC 的工程应用提供了借鉴意义。
提出了 MMC 调制技术的改进方向,对进一步的研究探索有积极意义。
关键词:模块化多电平换流器;调制技术;载波移相调制法;载波层叠调制;最近电平逼近调制;多电平SVPWM ;特定次谐波消除脉宽调制中图分类号:TM 46文献标志码# A文章编号# 2095-8188(2017)08-0043-05DOI : 10.16628/j . cnki . 2095-8188. 2017. 08. 011王 蕊(1993—),女,硕士研究生,研 究方向为电力电子 技术在电力系统中 的应用。
Review on Modulation Metliods for Modular Multi-level ConvertersWANG Rui 1, WANG Bin 2, WAN Jiexing 1(1. School of Electrical Engineering ,Southeast University ,Nanjing 210096,China ;2. China Ocean Engineering Baoshen Cable Co .,Ltd .,Nanjing 225100,China )Abstract : The topology and working principle ofmodular multi-level converter ( MMC ) were introduced andthe different modulation methods were introduced in detail . Next,it summarized the advantages and disadvantages of different modulation techniques and applications,providing a reference for the MMC ) s engineering application .At last , this paper put forward the improvement direction of MMC modulation technology ,significance for the further research and exploration .Key words : modular multi-level converter ( MMC ); modulation technique ; carrier phase shifted SPWM ( CPS -SPWM ); phase disposition PWM (PDPWM ); nearest level modulation (NLM ); multi-level space vector PWM ( SVPWM ); selective harmonic elimination PWM ( SHEPWM )步的研究成果,展现出良好的应用前景[1]。
Principles of Biochemistry 习题答案chapter 6
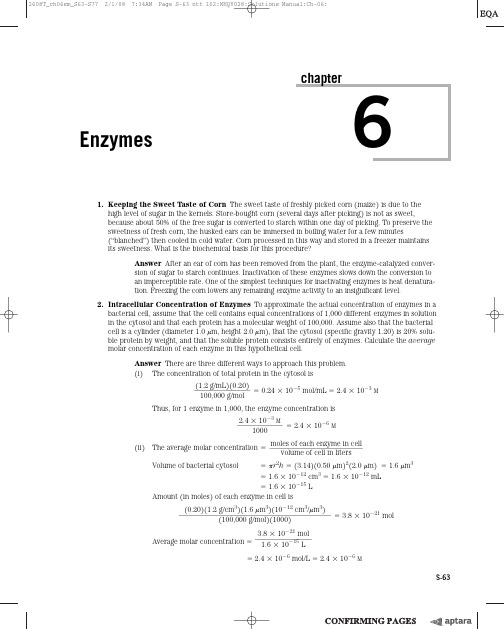
moles of each enzyme in cell volume of cell in liters 1.6 mm3
pr2h (3.14)(0.50 mm)2(2.0 mm) 1.6 10 12 cm3 1.6 10 12 mL 1.6 10 15 L Amount (in moles) of each enzyme in cell is (0.20)(1.2 g/cm3)(1.6 mm3)(10 12 cm3/mm3) (100,000 g/mol)(1000) 3.8 10
21
mol
Average molar concentration
3.8 10 21 mol 1.6 10 15 L 2.4 10
6
mol/L
2.4
10
6
M
S-63
2608T_ch06sm_S63-S77
2/1/08
7:34AM
Page S-64 ntt 102:WHQY028:Solutions Manual:Ch-06:
2608T_ch06sm_S63-S77
2/1/08
7:34AM
Page S-63 ntt 102:WHQY028:Solutions Manual:Ch-06:chpterEnzymes
6
1. Keeping the Sweet Taste of Corn The sweet taste of freshly picked corn (maize) is due to the high level of sugar in the kernels. Store-bought corn (several days after picking) is not as sweet, because about 50% of the free sugar is converted to starch within one day of picking. To preserve the sweetness of fresh corn, the husked ears can be immersed in boiling water for a few minutes (“blanched”) then cooled in cold water. Corn processed in this way and stored in a freezer maintains its sweetness. What is the biochemical basis for this procedure? Answer After an ear of corn has been removed from the plant, the enzyme-catalyzed conversion of sugar to starch continues. Inactivation of these enzymes slows down the conversion to an imperceptible rate. One of the simplest techniques for inactivating enzymes is heat denaturation. Freezing the corn lowers any remaining enzyme activity to an insignificant level. 2. Intracellular Concentration of Enzymes To approximate the actual concentration of enzymes in a bacterial cell, assume that the cell contains equal concentrations of 1,000 different enzymes in solution in the cytosol and that each protein has a molecular weight of 100,000. Assume also that the bacterial cell is a cylinder (diameter 1.0 mm, height 2.0 mm), that the cytosol (specific gravity 1.20) is 20% soluble protein by weight, and that the soluble protein consists entirely of enzymes. Calculate the average molar concentration of each enzyme in this hypothetical cell. Answer There are three different ways to approach this problem. (i) The concentration of total protein in the cytosol is (1.2 g/mL)(0.20) 100,000 g/mol 0.24 10
电气自动化专业外语翻译作业
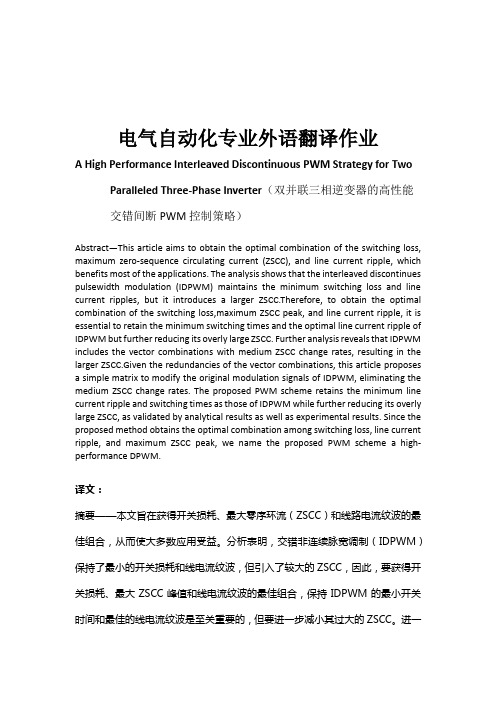
电气自动化专业外语翻译作业A High Performance Interleaved Discontinuous PWM Strategy for TwoParalleled Three-Phase Inverter(双并联三相逆变器的高性能交错间断PWM控制策略)Abstract—This article aims to obtain the optimal combination of the switching loss, maximum zero-sequence circulating current (ZSCC), and line current ripple, which benefits most of the applications. The analysis shows that the interleaved discontinues pulsewidth modulation (IDPWM) maintains the minimum switching loss and line current ripples, but it introduces a larger ZSCC.Therefore, to obtain the optimal combination of the switching loss,maximum ZSCC peak, and line current ripple, it is essential to retain the minimum switching times and the optimal line current ripple of IDPWM but further reducing its overly large ZSCC. Further analysis reveals that IDPWM includes the vector combinations with medium ZSCC change rates, resulting in the larger ZSCC.Given the redundancies of the vector combinations, this article proposes a simple matrix to modify the original modulation signals of IDPWM, eliminating the medium ZSCC change rates. The proposed PWM scheme retains the minimum line current ripple and switching times as those of IDPWM while further reducing its overly large ZSCC, as validated by analytical results as well as experimental results. Since the proposed method obtains the optimal combination among switching loss, line current ripple, and maximum ZSCC peak, we name the proposed PWM scheme a high-performance DPWM.译文:摘要——本文旨在获得开关损耗、最大零序环流(ZSCC)和线路电流纹波的最佳组合,从而使大多数应用受益。
药学英语Unit 6 Text A 注释及译文
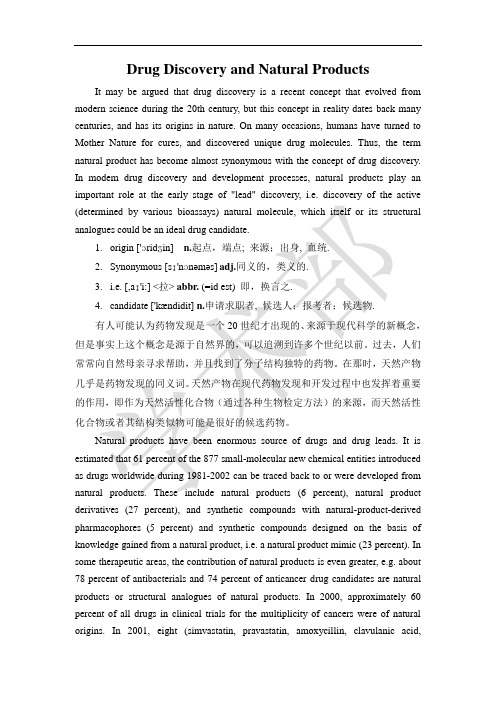
Drug Discovery and Natural Products It may be argued that drug discovery is a recent concept that evolved from modern science during the 20th century, but this concept in reality dates back many centuries, and has its origins in nature. On many occasions, humans have turned to Mother Nature for cures, and discovered unique drug molecules. Thus, the term natural product has become almost synonymous with the concept of drug discovery. In modem drug discovery and development processes, natural products play an important role at the early stage of "lead" discovery, i.e. discovery of the active (determined by various bioassays) natural molecule, which itself or its structural analogues could be an ideal drug candidate.1.origin ['ɔridʒin] n.起点,端点; 来源;出身, 血统.2.Synonymous [sɪ'nɔnəməs]adj.同义的,类义的.3.i.e. [,aɪ'i:] <拉> abbr. (=id est) 即,换言之.4.candidate ['kændidit] n.申请求职者, 候选人;报考者;候选物.有人可能认为药物发现是一个20世纪才出现的、来源于现代科学的新概念,但是事实上这个概念是源于自然界的,可以追溯到许多个世纪以前。
chap06 Signal Encoding Techniques 无线网络通信 英文课件

Multiple Frequency-Shift Keying (MFSK)
To match data rate of input bit stream, each output signal element is held for:
Ts=LT seconds
where T is the bit period (data rate = 1/T)
binary 1 binary 0
where f1 and f2 are offset from carrier frequency fc by equal but opposite amounts
Binary Frequency-Shift Keying (BFSK)
Less susceptible to error than ASK On voice-grade lines, used up to 1200bps Used for high-frequency (3 to 30 MHz)
error
s itA co 2fs it 1iM
f i = f c + (2i – 1 – M)f d f c = the carrier frequency f d = the difference frequency M = number of different signal elements = 2 L L = number of bits per signal element
So, one signal element encodes L bits
Multiple Frequency-Shift Keying (MFSK)
Total bandwidth required
2Mfd
移动通信(第六版)(章坚武)课件章 (4)
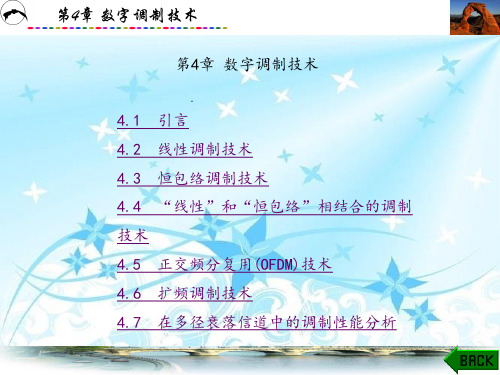
第4章 数字调制技术 图4-14 π/4-QPSK调制电路
第4章 数字调制技术 2. π/4-QPSK信号的解调 1) 基带差分检测(Baseband Differential Detection)
图4-15 基带差分检测电路
第4章 数字调制技术
第4章 数字调制技术
第4章 数字调制技术
第4章 数字调制技术 2)中频延迟差分检测(IFDifferentialDetection) 中频延迟差分检测电路如图4-16所示。
第4章 数字调制技术 图4-9 QPSK的相位关系图
第4章 数字调制技术 图4-10 OQPSK信号调制器框图
第4章 数字调制技术 图4-11 OQPSK的I、Q信道波形及相位路径
第4章 数字调制技术 图4-12 OQPSK相位关系图
第4章 数字调制技术
4.2.5 π/4-QPSK π/4-QPSK调制是对OQPSK和QPSK在实际最大相位变化
测方式的误比特率与比特能量噪声功率密度比(Eb/N0)之间的 关系式为
第4章 数字调制技术
在稳态高斯信道中,根据式(4-49)可作出π/4-QPSK基带 差分检测误码性能曲线如图4-19所示。它比实际的差分检测曲 线好2dB功率增益,比QPSK相干检测曲线差3dB功率增益。
在快瑞利衰落信道条件下,误码性能曲线如图4-20所示。 它是以多普勒频移fD作为参量所作的一组曲线。由图可见,当 fD=80Hz时,只要Eb/N0=26dB,可得误码率BER≤10-3,其性能 仍优于一般的恒包络窄带数字调制技术。
2Es Ts
cos2πfct
(i 1)
π 2
0≤t≤Ts i=1, 2, 3,
4
(4-
21)
光学频率梳的相干合成研究进展
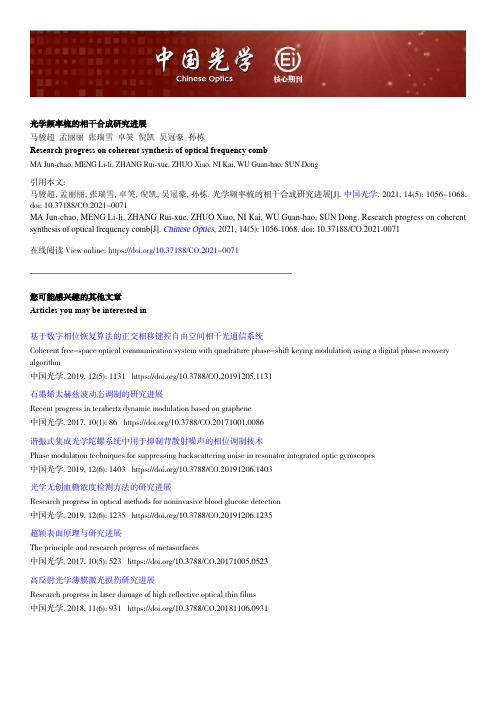
光学频率梳的相干合成研究进展马骏超 孟丽丽 张瑞雪 卓笑 倪凯 吴冠豪 孙栋Research progress on coherent synthesis of optical frequency combMA Jun-chao, MENG Li-li, ZHANG Rui-xue, ZHUO Xiao, NI Kai, WU Guan-hao, SUN Dong引用本文:马骏超,孟丽丽,张瑞雪,卓笑,倪凯,吴冠豪,孙栋. 光学频率梳的相干合成研究进展[J]. 中国光学, 2021, 14(5): 1056-1068. doi: 10.37188/CO.2021-0071MA Jun-chao, MENG Li-li, ZHANG Rui-xue, ZHUO Xiao, NI Kai, WU Guan-hao, SUN Dong. Research progress on coherent synthesis of optical frequency comb[J]. Chinese Optics, 2021, 14(5): 1056-1068. doi: 10.37188/CO.2021-0071在线阅读 View online: https:///10.37188/CO.2021-0071您可能感兴趣的其他文章Articles you may be interested in基于数字相位恢复算法的正交相移键控自由空间相干光通信系统Coherent free-space optical communication system with quadrature phase-shift keying modulation using a digital phase recovery algorithm中国光学. 2019, 12(5): 1131 https:///10.3788/CO.20191205.1131石墨烯太赫兹波动态调制的研究进展Recent progress in terahertz dynamic modulation based on graphene中国光学. 2017, 10(1): 86 https:///10.3788/CO.20171001.0086谐振式集成光学陀螺系统中用于抑制背散射噪声的相位调制技术Phase modulation techniques for suppressing backscattering noise in resonator integrated optic gyroscopes中国光学. 2019, 12(6): 1403 https:///10.3788/CO.20191206.1403光学无创血糖浓度检测方法的研究进展Research progress in optical methods for noninvasive blood glucose detection中国光学. 2019, 12(6): 1235 https:///10.3788/CO.20191206.1235超颖表面原理与研究进展The principle and research progress of metasurfaces中国光学. 2017, 10(5): 523 https:///10.3788/CO.20171005.0523高反射光学薄膜激光损伤研究进展Research progress in laser damage of high reflective optical thin films中国光学. 2018, 11(6): 931 https:///10.3788/CO.20181106.0931文章编号 2095-1531(2021)05-1056-13光学频率梳的相干合成研究进展马骏超1†,孟丽丽1,2†,张瑞雪3,卓 笑1,倪 凯4,吴冠豪3,孙 栋1,5 *(1. 北京大学 物理学院 量子材料科学中心,北京 100871;2. 北京科技大学 数理学院,北京 100083;3. 清华大学 精密仪器系 精密测试技术及仪器国家重点实验室,北京 100084;4. 清华大学 深圳国际研究生院 先进制造学部,广东深圳 518055;5. 量子物态协同创新中心,北京 100871)† 共同贡献作者摘要:光学频率梳(光频梳)具有脉冲宽度窄、频率精度高、频率梳齿稳定以及相干性好等优良的时频域特性,近年来成为超快激光技术及计量科学等领域的研究热点,并发展成为一种重要的科研工具。
利用模拟通信信道传输数字信号的方法
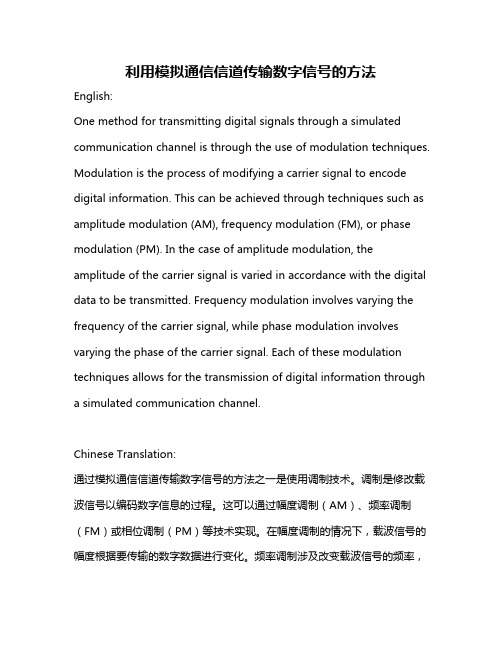
利用模拟通信信道传输数字信号的方法English:One method for transmitting digital signals through a simulated communication channel is through the use of modulation techniques. Modulation is the process of modifying a carrier signal to encode digital information. This can be achieved through techniques such as amplitude modulation (AM), frequency modulation (FM), or phase modulation (PM). In the case of amplitude modulation, the amplitude of the carrier signal is varied in accordance with the digital data to be transmitted. Frequency modulation involves varying the frequency of the carrier signal, while phase modulation involves varying the phase of the carrier signal. Each of these modulation techniques allows for the transmission of digital information through a simulated communication channel.Chinese Translation:通过模拟通信信道传输数字信号的方法之一是使用调制技术。
智能家居医疗保健中英文对照外文翻译文献

智能家居医疗保健中英⽂对照外⽂翻译⽂献智能家居医疗保健中英⽂对照外⽂翻译⽂献(⽂档含英⽂原⽂和中⽂翻译)原⽂:Foreign LiteratureIncreasing an individual’s quality of life via their intelligent homeThe hypothesis of this project is: can an individual’s quality of life be increased by integrating “intelligent technology”into their home environment. This hypothesis is very broad, and hence the researchers will investigate it with regard to various, potentially over-lapping, sub-sections of the population. In particular, the project will focus on sub-sections with health-care needs, because it is believed that these sub-sections will receive the greatest benefit from this enhanced approach to housing. Two research questions flow from this hypothesis: what are the health-care issues that could be improved via “intelligent housing”, and what are the technological issues needing to be solved to allow “intelligent housing”to be constructed?While a small number of initiatives exist, outside Canada, which claim to investigate this area, none has the global vision of this area. Work tends to be in small areas with only a limited idea of how the individual pieces contribute towards a greater goal. This project has a very strong sense of what it is trying to attempt, and believes that without this global direction the other initiatives will fail to address the large important issues described within various parts of this proposal, and that with the correct global direction the sum of the parts will produce much greater rewards than the individual components. This new field has many parallels with the field of business process engineering, where many products fail due to only considering a sub-set of the issues, typically the technology subset. Successful projects and implementations only started flow when people started to realize that a holistic approach was essential. Thisholistic requirement also applies to the field of “smart housing”; if we genuinely want it to have benefit to the community rather than just technological interest. Having said this, much of the work outlined below is extremely important and contains a great deal of novelty within their individual topics.Health-Care and Supportive housing:To date, there has been little coordinated research on how “smart house”technologies can assist frail seniors in remaining at home, and/or reduce the costs experienced by their informal caregivers. Thus, the purpose of theproposed research is to determine the usefulness of a variety of residential technologies in helping seniors maintain their independence and in helping caregivers sustain their caring activities. The overall design of the research is to focus on two groups of seniors. The first is seniors who are being discharged from an acute care setting with the potential for reduced ability to remain independent. An example is seniors who have had hip replacement surgery. This group may benefit from technologies that would help them become adapted to their reduced mobility.The second is seniors who have a chronic health problem such as dementia and who are receiving assistance from an informal caregiver living at a distance. Informal caregivers living at a distance from the cared-for senior are at high risk of caregiver burnout. Monitoring the cared-for senior for health and safety is one of the important tasks done by such caregivers. Devices such as floor sensors (to determine whether the senior has fallen) and access controls to ensure safety from intruders or to indicate elopement by a senior with dementia could reduce caregiver time spent commuting to monitor the senior. For both samples, trials would consist of extended periods of residence within the ‘smart house’. Samples of seniors being discharged from acute care would be recruited from acute care hospitals. Samples of seniors being cared for by informal caregivers at a distance could be recruited through dementia diagnosis clinics or through request from caregivers for respite.Limited amounts of clinical and health service research has been conducted upon seniors (with complex health problems) in controlled environments such as that represented by the“smart house”. For example, it is known that night vision of the aged is poor but there is very little information regarding the optimum level of lighting after wakening or for night activities.Falling is a major issue for older persons; and it results in injuries, disabilities and additional health care costs. For those with dementing illnesses, safety is the key issue during performance of the activities of daily living (ADL). It is vital for us to be able to monitor where patients would fall during ADL. Patients and caregivers activities would be monitored and data will be collected in the following conditions.Projects would concentrate on sub-populations, with a view to collecting scientific data about their conditions and the impact of technology upon their life styles. For example:Persons with stable chronic disability following a stroke and their caregivers: to research optimum models, types and location of various sensors for such patients (these patients may have neglect, hemiplegia, aphasia and judgment problems); to research pattern of movements during the ambulation, use of wheel chairs or canes on various type of floor material; to research caregivers support through e-health technology; to monitor frequencies and location of the falls; to evaluate the value of smart appliances for strokepatients and caregivers; to evaluate information and communication technology set up for Tele-homecare; to evaluate technology interface for Tele-homecare staff and clients; to evaluate the most effective way of lighting the various part of the house; to modify or develop new technology to enhance comfort and convenience of stroke patients and caregivers; to evaluate the value of surveillance systems in assisting caregivers.Persons with Alzheimer’s disease and their caregivers: to evaluate the effect of smart house (unfamiliar environment) on their ability to conduct self-care with and without prompting; to evaluate their ability to use unfamiliar equipment in the smart house; to evaluateand monitor persons with Alzheimer’s disease movement pattern; to evaluate and monitor falls or wandering; to evaluate the type and model of sensors to monitor patients; to evaluate the effect of wall color for patients and care givers; to evaluate the value of proper lighting.Technology - Ubiquitous Computing:The ubiquitous computing infrastructure is viewed as the backbone of the “intelligence”within the house. In common with all ubiquitous computing systems, the primary components with this system will be: the array of sensors, the communication infrastructure and the software control (based upon software agents) infrastructure. Again, it is considered essential that this topic is investigated holistically.Sensor design: The focus of research here will be development of(micro)-sensors and sensor arrays using smart materials, e.g. piezoelectric materials, magneto strictive materials and shape memory alloys (SMAs). In particular, SMAs are a class of smart materials that are attractive candidates for sensing and actuating applications primarily because of their extraordinarily high work output/volume ratio compared to other smart materials. SMAs undergo a solid-solid phase transformation when subjected to an appropriate regime of mechanical and thermal load, resulting in a macroscopic change in dimensions and shape; this change is recoverable by reversing the thermo mechanical loading and is known as a one-way shape memory effect. Due to this material feature, SMAs can be used as both a sensor and an actuator. A very recent development is an effort to incorporate SMAs in micro-electromechanical systems (MEMS) so that these materials can be used as integral parts of micro-sensors and actuators. MEMS are an area of activity where some of the technology is mature enough for possible commercial applications to emerge. Some examples are micro-chemical analyzers, humidity and pressure sensors, MEMS for flow control, synthetic jet actuators and optical MEMS (for the next generation internet). Incorporating SMAs in MEMS is a relatively new effort in the research community; to the best of our knowledge, only one group (Prof. Greg Carman, Mechanical Engineering, University of California, Los Angeles) has successfully demonstrated the dynamic properties of SMA-based MEMS. Here, the focus will be to harness the sensing and actuationcapabilities of smart materials to design and fabricate useful and economically viable micro-sensors and actuators.Communications: Construction and use of an “intelligent house”offers extensive opportunities to analyze and verify the operation of wireless and wired home-based communication services. While some of these are already widely explored, many of the issues have received little or no attention. It is proposed to investigate the following issues:Measurement of channel statistics in a residential environment: knowledge of the indoor wireless channel statistics is critical for enabling the design of efficient transmitters and receivers, as well as determining appropriate levels of signal power, data transfer rates, modulation techniques, and error control codes for the wireless links. Interference, channel distortion, and spectral limitations that arises as a result of equipment for the disabled (wheelchairs, IV stands, monitoring equipment, etc.) is of particular interest. Design, analysis, and verification of enhanced antennas for indoor wireless communications. Indoor wireless communications present the need for compact and rugged antennas. New antenna designs, optimized for desired data rates, frequency of operation, and spatial requirements, could be considered.Verification and analysis of operation of indoor wireless networks: wireless networking standards for home automation have recently been commercialized. Integration of one or more of these systems into the smarthouse would provide the opportunity to verify the operation of these systems, examine their limitations, and determine whether the standardsare over-designed to meet typical requirements.Determination of effective communications wiring plans for “smart homes.”: there exist performance/cost tradeoffs regarding wired and wireless infrastructure. Measurement and analysis of various wireless network configurations will allow for determination of appropriate network designs. Consideration of coordinating indoor communication systems withlarger-scale communication systems: indoor wireless networks are local to the vicinity of the residence.There exist broader-scale networks, such as the cellular telephone network, fixed wireless networks, and satellite-based communication networks. The viability and usefulness of compatibility between these services for the purposes of health-care monitoring, the tracking of dementia patients, etc needs to be considered.Software Agents and their Engineering: An embedded-agent can be considered the equivalent of supplying a friendly expert with a product. Embedded-agents for IntelligentBuildings pose a number of challenges both at the level of the design methodology as well as the resulting detailed implementation. Projects inthis area will include:Architectures for large-scale agent systems for human inhabitedenvironment: successful deployment of agent technology inresidential/extended care environments requires the design of new architectures for these systems. A suitable architecture should be simple and flexible to provide efficient agent operation in real time. At the same time, it should be hierarchical and rigid to allow enforcement of rules and restrictions ensuring safety of the inhabitants of the building system. These contradictory requirements have to be resolved by designing a new architecture that will be shared by all agents in the system.Robust Decision and Control Structures for Learning Agents: to achievelife-long learning abilities, the agents need to be equipped with powerful mechanisms for learning and adaptation. Isolated use of some traditional learning systems is not possible due tohigh-expected lifespan of these agents. We intend to develop hybrid learning systems combining several learning and representation techniques in an emergent fashion. Such systems will apply different approaches based on their own maturity and on the amount of change necessary to adapt to a new situation or learn new behaviors. To cope with high levels ofnon-determinism (from such sources as interaction with unpredictable human users), robust behaviors will be designed and implemented capable of dealing with different types of uncertainty (e.g. probabilistic and fuzzy uncertainty) using advanced techniques for sensory and data fusion, and inference mechanisms based on techniques of computational intelligence. Automatic modeling of real-world objects, including individual householders: The problems here are: “the locating and extracting”of information essential for representation of personality and habits of an individual; development of systems that “follow and adopt to”individual’s mood and behavior. The solutions, based on data mining and evolutionary techniques, will utilize: (1) clustering methods, classification tress and association discovery techniques for the classification and partition of important relationships among different attributes for various features belonging to an individual, this is an essential element in finding behavioral patterns of an individual; and (2) neuro-fuzzy and rule-based systems with learningand adaptation capabilities used to develop models of an individual’s characteristics, this is essential for estimation and prediction of potential activities and forward planning.Investigation of framework characteristics for ubiquitous computing: Consider distributed and internet-based systems, which perhaps have the most in common with ubiquitous computing, here again, the largest impact is not from specific software engineering processes,but is from available software frameworks or ‘toolkits’, which allow the rapid construction and deployment of many of the systems in these areas. Hence, it is proposed that the constructionof the ubiquitous computing infrastructure for the “smart house”should。
- 1、下载文档前请自行甄别文档内容的完整性,平台不提供额外的编辑、内容补充、找答案等附加服务。
- 2、"仅部分预览"的文档,不可在线预览部分如存在完整性等问题,可反馈申请退款(可完整预览的文档不适用该条件!)。
- 3、如文档侵犯您的权益,请联系客服反馈,我们会尽快为您处理(人工客服工作时间:9:00-18:30)。
= S Ac [1 + m(t ) ] cos ( 2π f c t ) , AM BAM =2 f m , PAM 1 2 2 = Ac 1 + 2 m(t ) + m (t ) 2
Demodulation of AM: non-coherent envelope detector and coherent product detector.
Unipolar NRZ
Bipolar RZ
Manchester NRZ
6.4 Pulse Shaping Techniques ISI: rectangular pulses are passed through a band-limited channel, pulses will spread in time, and the pulse for each symbol will smear into the time intervals of succeeding symbols. To minimize ISI: increase the channel bandwidth and reduce the modulation bandwidth. Out-of-band radiation in the adjacent channel in a mobile radio system should generally be 40 dB to 80 dB below that in the desired pass-band.
FM vs. AM FM has better noise immunity. An FM signal is a constant envelop signal, FM exhibits a capture effect characteristic. Constant envelop allows efficient Class C power amplifiers for RF power amplifica. To require a wider frequency band to obtain the advantages of reduced noise and capture effect. FM transmitter and receiver equipment is also more complex. Nhomakorabea
Since it is difficult to directly manipulate the transmitter spectrum at RF frequencies, spectral shaping is done through base-band or IF processing. There are a number of well know pulse shaping techniques which are used to simultaneously reduce the ISI and the spectral width of a modulated digital signal.
模 拟 调 制
数 字 调 制
常用调制方式 常规双边带调幅(AM) 抑制载波双边带调幅(DSB) 线性调制 单边带调幅(SSB) 残留边带调幅(VSB) 频率调制(FM) 非线性调制 相位调制(PM) 线性 相移键控(BPSK,DPSK,QPSK 等) 频移键控(BFSK) 非线性 最小频移键控(MSK) 多进制相移键控(MPSK) 线性和非线性联合 多进制正交幅度调制(QAM) 多进制频移键控(MFSK)
6.2 Digital Modulation-An Overview A desirable modulation scheme provides low BER at low received SIR, performs well in multipath and fading conditions, occupies a minimum of bandwidth, and is easy and cost-effective to implement. The performance of a modulation scheme is often measured by pow er efficiency and bandw idth efficiency .
Power efficiency: ability to preserve the fidelity of the digital message at low power levels, ηp (Eb/N0). Bandwidth efficiency: ability to accommodate data within a limited bandwidth, ηb=R/B bps/Hz. The system capacity is directly to bandwidth efficiency of the modulation. Shannon’s bound applies for AWGN . C S = = log 2 1 + η B max B N
The absolute bandwidth of a signal: has a non-zero power spectral density. The null-to-null bandwidth: is equal to the width of main spectral lobe. The half-power (3dB ) bandwidth: PSD has drop to half power, or 3dB below the peak value. Another commonly used method to specify bandwidth is to state that everywhere outside the specified band, the PSD is below a certain stated level.(45dB to 60dB attenuation).
6.4.1 Nyquist Criterion for ISI Cancellation Nyquist was the first to solve the problem of overcoming ISI while keeping the transmission bandwidth low. The effect of ISI could be completely nullified if the overall response of the communication system is designed so that at every sampling instant at the receiver, the response due to all symbol except the current symbol is equal to zero.
t
∆f , 调相指数:β p = kθ Am = ∆θ 调频指数:β f = = W W Carson公式:BT = BT = f 2 ( β f + 1) f(上边带), 2∆(下边带) m
FM detection: frequency discriminators.
AM: the amplitude of a high frequency carrier signals is varied with the instantaneous amplitude of the modulating message signal.
Chapter6. Modulation Techniques for Mobile Radio
To encode information from a message source in a manner suitable for transmission. To translate a baseband message signal to a bandpass signal at very high frequencies. Modulated signal (bandpass signal), modulating signal (baseband message signal ). To vary the amplitude, phase, or frequency of a high frequency carrier. Analog modulation (1G mobile radio systems) Digital modulation (current and future systems)
6.3 Line coding Digital baseband signals often use line codes to provide particular spectral characteristics of a pulse train. The most common codes for mobile communication are RZ, NRZ, and Manchester codes. RZ codes: pulse returns to zero within every bit period. This leads to spectral widening, but improves timing sync.
6.1 Analog modulation techniques FM: frequency is varied by modulating message signals.
cos 2π f t + 2π k π θ S FM Ac f t t A cos 2 = + = ( ) c c c f k f 频偏常数(Hz/V) S PM Ac cos 2π f c t + kθ m ( t ) k f Am kθ 相移常数(rad/V) η η m d ( ) ∫-∞