astrocyte calcium-glia
ALS
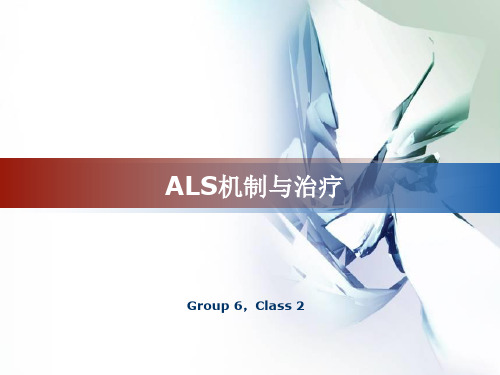
classification:
1.中枢: 星形胶质细胞(astrocyte)、 大胶质细胞(macroglia) 少突胶质细胞(oligodendrocyte)、 小胶质细胞(microglia) 纤维性星形胶质细胞 原浆性星形胶质细胞 2.周围: 神经膜细胞(Schwann cell,雪旺细胞) 卫星细胞(satellite cell ) The main cell relating to ALS are astrocyte and microglia.
ALS机制与治疗
Group 6,Class 2
ALS
Contents
1
2 3
Introduction Mechanism Treatment
Company Logo
ALS
Introduction
Amyotrophic lateral sclerosis,ALS: 一种进行性发展的致死性神经系统变性疾 病,选择性累及脊髓前角细胞和大脑皮质 运动神经元。
Company Logo
ALS
Introduction
Manifestation: upper and lower motor neuron injury. Asymmetry myasthenia and amyotrophic from the distal part of the limbs. Result: Die within 3-5 years,die of bulbar Байду номын сангаасaralysis,respiratory muscular paralysis and pulmonary infection.
d. bacteria and virus infection:
第2章-神经元与胶质细胞-PPT

按递质分类
5-HT能神经元 NE能神经元 DA能神经元 Ach能神经元 …….
按电生理特性分类
兴奋性神经元 抑制性神经元
2.1.4 神经元间的联系
简单回路
辐散(divergence) 聚合(convergence) 链锁状和环状
不同层次 神经环路
不同核团或皮层脑区和之间的长投射纤维 同一核团或脑区的局部环路 相邻神经元不同成分间的微环路
小脑内局部神经元回路
1:藓苔纤维 2:攀缘纤维 3:小脑深部核团细胞 4:颗粒细胞 5:高尔基细胞 6:浦肯野细胞 7:篮状细胞 8:星状细胞 9:平行纤维 黑色细胞均为抑制性神经元
2.1.5 神经元特有蛋白 和代谢特点
纤维性星型胶质细胞 原浆性星型胶质细胞
多分布于脑和脊髓的白质,突起 细长,分支少,“蜘蛛细胞”, 富含胶质丝
多分布于灰质,突起粗短,分 支多,“苔状细胞”,较少胶 质丝
特殊的星形胶质细胞 Bergmann胶质细胞: 小脑皮质,原浆性为主 Muller胶质细胞:视网膜 垂体细胞:脑垂体后叶 伸展细胞:正中隆起
分类
大胶质细胞
中枢胶质细胞 (macroglia)
星形胶质细胞 (astrocyte) 少突胶质细胞(oligodendrocyte)
外周胶质细胞
小胶质细胞
(microglia)
施万细胞
(Schwann cell)
室管膜细胞 (ependymocyte)
脉络丛细胞 (choroidal
epithelial cell)
• 脑毛细血管表面85%-99%被其足板覆盖 神经元
阿拉丁羟乙基纤维素

阿拉丁羟乙基纤维素
阿拉丁羟乙基纤维素是一种常用的增稠剂和胶凝剂,被广泛应用于食品、药品、化妆品等行业中。
其化学名为羟乙基纤维素,英文名为Hydroxyethyl Cellulose,缩写为HEC。
阿拉丁羟乙
基纤维素的主要特点是水溶性良好,能够在水中形成清澈透明的胶体溶液。
阿拉丁羟乙基纤维素在食品中的应用包括作为增稠剂、稳定剂、乳化剂等。
它可以增加食品的黏性和口感,改善食品的质地,提高乳化性能,增强稳定性。
在药品中,阿拉丁羟乙基纤维素可以用作药片的胶囊剂、乳化剂和混悬剂,能够调整药物的缓释性能、改善口感、增加稳定性。
在化妆品中,阿拉丁羟乙基纤维素常用作凝胶剂和稠化剂,能够改善产品的质地和稠度,增加粘度和黏性,提高使用体验。
总之,阿拉丁羟乙基纤维素在各个行业中都有广泛的应用,能够起到增稠、胶凝、乳化等作用,提高产品的品质和稳定性。
火星生命探测中一种潜在的生物标志物磷酸盐
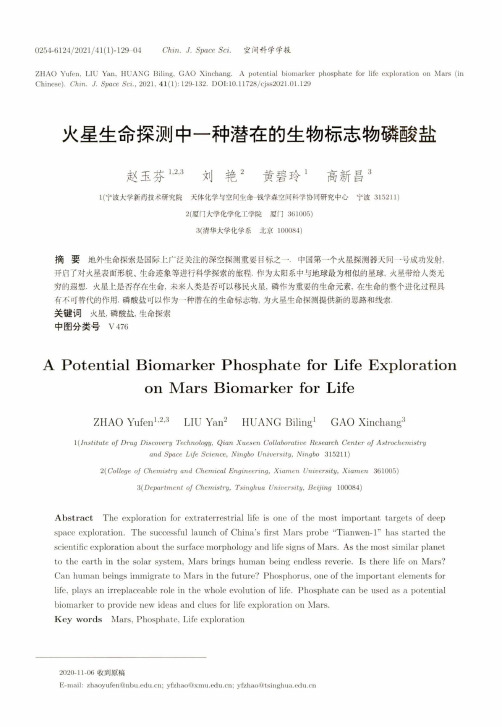
0254-6124/2021/41(1)-129-04 Chin. J. Space Sci.空间科学学报ZHAO Yufen. LIU Yan, HUANG Biling, GAO Xinchang. A potential biomarker phosphate for life exploration on Mars (in Chinese). Chin. J. Space Sci, 2021, 41(1): 129-132. D01:10.11728/cjss2021.01.129火星生命探测中一种潜在的生物标志物磷酸盐赵玉芬1>2’3刘艳2黄碧玲1高新昌31(宁波大学新药技术研究院天体化学与空间生命#学森空间科学协同研究中心宁波315211)门大学化学化工学院ffl门361005)3(清华大学化学系北京100084)f商要地外生命探索是国际上广泛关注的深空探测重要目标之一.中国第一个火星探测器天问-号成功发射,开启了对火星表面形貌、生命迹象等进行科学探索的旅程.作为太阳系中与地球最为相似的星球.火星带给人类无 穷的遐想.火星上是否存在生命,未来人类是否可以移民火星,磷作为重要的生命元素,在生命的整个进化过程具t不可替代的作用.磷酸盐可以作为-种潜在的生命标志物,为火星生命探测提供新的思路和线索.关键词火星,磷酸盐,生命探索中图分类号V476A P oten tial Biom arker P h osp h ate for Life E xplorationon M ars Biom arker for LifeZHAO Yufen1’2,3LIU Yan2HUANG Biling1GAO Xinchang3\[Instit,ute of Drug Discovery Technology, Qian Xuesen Collaborative Research Center of Astrochemistryand Space Life Science, Ningbo University, Ningbo315211)2(College of Chemistry and Chemical Engineering, Xiamen University, Xiamen361005)^(Department of Chemistry, Tsinghua University, Beijing100084)Abstract T h e e x p l o r a t i o n f o r e x t r a t e r r e s t r i a l life is o n e o f t h e m o s t i m p o r t a n t t a r g e t s o f d e e ps p a c e e x p l o r a t i o n.T h e s u c c e s s f u l l a u n c h o f C h i n a’s first M a r s p r o b e“T i a n w e n-1”h a s s t a r t e d t h e s c i e n t i f i c e x p l o r a t i o n a b o u t t h e s u r f a c e m o r p h o l o g y a n d life s i g n s o f M a r s.A s t h e m o s t s i m i l a r p l a n e t t o t h e e a r t h i n t h e s o l a r s y s t e m,M a r s b r i n g s h u m a n b e i n g e n d l e s s r e v e r i e.I s t h e r e life o n M a r s?C a n h u m a n b e i n g s i m m i g r a t e t o M a r s i n t h e f u t u r e?P h o s p h o r u s,o n e o f t h e i m p o r t a n t e l e m e n t s f o rlife, p l a y s a n i r r e p l a c e a b l e r o l e i n t h e w h o l e e v o l u t i o n o f life. P h o s p h a t e c a n b e u s e d a s a p o t e n t i a lb i o m a r k e r t o p r o v i d e n e w i d e a s a n dc l u e s f o r life e x p l o r a t i o n o n M a r s.Key words M a r s,P h o s p h a t e,L i f e e x p l o r a t i o n2020-11-06收到原稿E-mail: *****************.cn;**************.cn;*******************.cnDNARNABaseOHBaseOH OH〇引言2020年7月23日12时41分,在中国文昌航天 发射场,长征五号遥四运载火箭将中国第一个火星探 测器天问一号成功发射.随着天问一号直刺苍穹,中 国开启了对火星表面形貌、物质成分、生命迹象等进 行科学探索的旅程.1火星上是否存在生命2019年度诺贝尔物理学奖授予了吉姆■皮布尔 斯(Jam es P eeb les )、米歇尔.麦耶(M ichel M a y o r)和 迪迪埃.奎洛兹(D idier Q u e lo z )三位科学家,以表彰 他们在宇宙物理学和太阳系外行星领域做出的贡献. 迄今在银河系中已发现了 4000多颗系外行星,这些 系外行星的发现改变了地球在宇宙中的地位.这些 行星中有类似地球的岩石行星,地球在宇宙中是否独 一无二,其他行星中是否也存在生命尚未可知.火星上是否有生命存在对于人类未来在火星建 立基地,进行火星移民有着非常重要的意义.据中国 古籍中记载,火星被古人称为“荧惑”,意为不详之星, 而西方则认为火星是罗马战神,通常被称为红色星 球.火星是离太阳第四近的行星,是太阳系四颗类地 行星之一.科学家们发现在太阳系中火星是与地球 最为相似的星球.首先,火星所处轨道接近太阳系的 宜居地带,尽管现在的火星是一个干燥、寒冷、贫瘠 的星球,却可能在以前或者现在正孕育着生命;其次, 火星的地表环境砾石遍布,北部是平原而南部是布 满陨石坑的高地,其地表环境特征与地球极为相似; 第三,目前发现火星上存在少量水,并在火星盖尔陨 石坑内发现富含矿物盐的沉积物;第四,火星大气层130中存在甲烷,构成甲烷的碳、氢元素是地球生命出现 的基础.因此,火星上具备了与生命存在相关的基本 条件.但是,科学家们尚未发现火星上生命存在的标 记物,无法确认火星上是否有生命存在.2磷是遗传物质不可替代的基本元素生命由核酸、蛋白质等物质组成.遗传信息的传 递遵循遗传学中心法则,即遗传物质贮存于脱氧核糖 核酸(D NA ), D NA进行自我复制.将遗传信息传递给子代D N A ,子代D NA 经转录过程将遗传信息传递给核糖核酸(R N A ), R NA在核糖体中经翻译过程形成生命活动的主要承担者——蛋白质,从而将遗传 信息传递给了蛋白质.磷酸二酯键是构成DN A 和R N A的基本骨架(见图1),磷在核酸中的含量为9%. D N A作为遗传物质,要求其有足够的稳定性,遗传信息才能长期保存并代代传递.而磷在保证D N A稳定性方面起到了关键性作用.1987年W e s t h e i m e r W研究提出:DN A的半衰期为1〇5年;当由硫替代磷酸二酯键中的磷时,其 半衰期为2.7h ;当由硅或砷替代磷时其半衰期则仅 为1〜2 m i n ;而由矾替代磷时极其不稳定,几乎立即 水解.另外,磷酸二酯键及其负电荷性质保护了D N A不被水解,并且使其保留于细胞内而不会穿过磷脂细胞膜.由此可见,遗传物质DN A中的磷是不可替代的.因此,磷元素的存在也应是行星生命探索的必要 条件之一.地球上最古老的蛋白是37亿年前产生的AT P结合蛋白其次是磷转移蛋白.二者在地球上出现氧气之前便已存在.在地球早期海洋中,金属F e , Mn,Chin . J . Space Sci .空间科学学报 2021,41(1)〇|o LV O—=p -—o -io .i-〇l p —〇二〇〇图1D N A 与R N A 结构片段F ig. 1D N A a n d R N A s tru c tu r a l fra g m e ntsC o, Ni含量较高.这些金属可以催化偏三磷酸钠与腺苷反应,将腺苷转化为A T P l '而A T P可以诱导A T P 结合蛋白的产生.生物体的生命活动都必须 有AT P的参与.人体每天可以产生并消耗的AT P量与自身体重相当ATP结合盒转运体(A T P-b i n d i n gc a s s e t t e t r a n s p o r t e r , A B C转运体)是一类A T P结合蛋白,其利用AT P水解释放的能量执行转运细胞内外物质的功能.保证细胞营养物质的充 足与代谢产物的清除.A B C转运体若发生功能障碍,将V 某些疾病的发生密切相关,如阿尔兹海默症、谷固醇血症等【5】.由此可见,磷元素是生命体必不可少的基本元 素.是不可替代的生命元素.在原始生命的形成过程 中具有重要作用.3地球上的磷矿生物沉集中国主要磷矿形成于距今6.5亿至5.5亿年的震 旦纪晚期及寒武纪成矿年代,当时中国长江流域的四 川、陕西、贵州、云南、湖北等省还是一片汪洋大海. 古称扬子古海.气候温和、阳光充足.繁殖有大量的 藻类.扬子古海的磷矿源于海洋中的生物遗骸.经 过1亿年,蓝绿藻在海洋中沉积了超过105亿吨的 胶磷矿(平均每年沉积约100吨),占中国磷资源总量 的75%.因此.磷酸盐可能是生物的遗骸,应该是很 好的生命标志物.4火星上_的探测基丁-磷元素对生命的重要意义.1957年度的诺 贝尔化学奖获得者L o r d (A l e x a n d e r R ) To d d教授于1981年在日本大阪召开的一次国际会议上做了 一个主旨为:“哪里有生命,哪里就有憐”的精彩报 告,并提出••我猜想,如果宇宙的其他地方有生命存 在的话,那么极有可能是发生在具有充足磷元素的星球上”161.因此.磷酸盐是生命探索的一种潜在标志 物.对地外生命的探索.也需探究地外星球上是否有 憐的存在.2〇13 年 A^u r e G e o s c i e n c e发表了 A d c o c k 等丨的研究成果.他们认为.通过勇气号火星探测车的a 粒子X 射线光谱法测到火星表面有磷.火星上的磷赵玉芬等:火星生命探测中一种潜在的生物标志物磷醆盐不同于地球.地球上的磷矿石主要存在形式是氟磷 灰石C a 5(P 04)3F ,而氣离子对生物体是有毒性的.因 此,炼磷矿过程中需将氟离子去除,不含氟离子的磷 肥才可用尹促进农作物的生长.而火星磷矿石的主 要存在形式是氯磷灰石C a 5(P 04)3C I ,氯离子是与生 命体共存的.是生物体可以利用的基本元素.通过对 矿石的溶解速率与溶解度分别进行比较分析,发现氯 磷灰石释放磷的速率远快于氟磷灰石.火星上水-岩 相互作用时.磷酸盐的释放速率是地球上的45倍.早 期火星上潮湿环境中的磷酸盐浓度可能是早期地球 上的2倍这些都表明火星上的磷酸盐可利用性 高于地球.5火星上的磷是否来自生物氧存在三种稳定同位素:160. 170, 180.三者在 地球圈的含量分别为99.759%, 0.037%和0.204%.磷 酸根P〇i 广中的氧原子16〇对非生物过程的同位素交换有很强的抵抗力,即在没有生物或酶参与下.p〇r与其他物质发生氧同位素交换很慢.实验证据表明,羟基磷灰石来自骨骼和牙齿的磷酸根,其180 含量高,说明同位素可以快速交换.而非生物的磷矿 石和花岗石18〇含量低[8,91.生物几乎参与了磷循环的每个过程.酶是细胞活 性的指示物.酶催化的磷酸盐与水分子之间的氧同位 素交换表明生命的存在.L i a n g 等11U 1和B l a k e等I 11)提出,P 18〇疒的<518〇p 值可能提供了存在地外生命的重要生物特征.如果将火星上的磷矿石带回地球, 那么磷酸根P 18〇〗_的<518O p 值可以作为火星生命 探索的标志物.<518〇p 的定义I 11]为Q x 1_.(1)\ "VSMOWf18〇式中,"R sample 为样品中的比值,尺VSMOW 为维也纳标准平均海水(V i e n n a S t a n d a r d Me a nO c e a n18〇W a t e r, VS M O W)中元石的比值.因此,若能知道宇宙样品中磷酸盐中氧同位素f 8〇P 原始值,将样品的测量值W 该原始值进行 比较,即可获知该样品中的磷是否曾被生物利用,便 可探测生命是否存在.1316火星上的磷可否被生物利用火星上的磷水溶性很好,但是火星上的盐为高氯酸盐,地球上的生物不能直接利用,必须将高氯酸盐 还原分解为氯离子才能实现生物利用.例如,可以利 用耐辐射的特殊微生物将其还原分解为氯离子,并进 一步利用多种功能微生物将火星上的磷、氮及钾解 离出来.氮及钾对地球上的植物仍是必须的.可以设 想将多种极端生物,如地衣、水熊虫、嗜冷菌、嗜压 菌等有机结合成一个小型生态圈,实现对火星土壤及 磷资源的有效利用.虽然上面的描述仅仅是设想,但 是理论上是可行的,值得潜心尝试研究,以期为今后 的“火星移民”提供重要的理论支撑及实验验证.7展望由于火星与地球相似并具备一些适宜的条件,其可能是一个潜在的可居住环境.人类若考虑对外星 球进行移民,火星将是人类的首选星球.但是,火星 对人类来说充满了未知,火星过去是否有生命存在. 磷是否来自火星生物遗骸,地球上的生命能否利用火 星上的磷及其他原位资源来生存,这些重要课题亟待 人类去研究探索,并激发人类为之不懈努力.天问一 号的成功发射,必将为中国的火星探测乃至深空探测 的研究带来前所未有的惊喜和收获.参考文献[1] WESTHEIMER F H. Why nature chose phosphates [J].Science, 1987, 235:1173-1178132[2] KIM K M, QIN T, JIANG Y Y, et al. Protein domainstructure uncovers the origin of aerobic metabolism and the rise of planetary oxygen [J ].2012,20:67-76[3] CHENG C, FAN C, WAN R, et al. Phosphorylation of adenosine with trimetaphosphate under simulated pre- biotic conditions [J]. Orig. Life Evol. Biosph., 2002, 32:219-224[4] TORNROTH-HORSEFIELD S, NEUTZE R. Opening and closing the metabolite gate [J]. Proc. Natl. Acad. Sci., 2008, 105:19565-19566[5] TARLING E J, DE AGUIAR VALLIM T Q, EDWARDS P A. Role of ABC transporters in lipid transport and human disease [J]. Trends Endocrinol. Metab.^ 2013, 24:342-350[6] TODD L. Where There's Life, There's Phosphorus [M ]. Tokyo: Japan Sciencs Society Press, 1981:275-279[7] ADCOCK C T, HAUSRATH E M, FORSTER P M. Readily available phosphate from minerals in early aqueous environments on Mars[J]. Nat. Geosci., 2013. 6:824- 827[8] SCHWARTZ A W. Phosphorus in prebiotic chemistry [J].Philos. Trans. R. Soc. Lond. B. Biol. Sci., 2006, 361: 1743-1749[9] LECUYER C, GRANDJEAN P, SHEPPARD S M F. Oxygen isotope exchange between dissolved phosphate and water at temperatures ( 135°C: inorganic versus biological fractionations [J]. Geochim. Cosmochim. Ac., 1999, 63:855-862[10] LIANG Y, BLAKE R E. Oxygen isotope fractionation between apatite and aqueous-phase phosphate: 20~45°C [J]. Chem. Geol, 2007, 238: 121-133[11] BLAKE R E, ALT J C, MARTINI A M. Oxygen isotoperatios of PO 4: an inorganic indicator of enzymatic activity and P metabolism and a new biomarker in the search for life[J]. Proc. Natl. Acad. Sci., 2001. 98:2148-2153Chin . J . Space Sci .空间科学学报 2021, 41(1)。
神经元和神经胶质细胞-胶质细胞
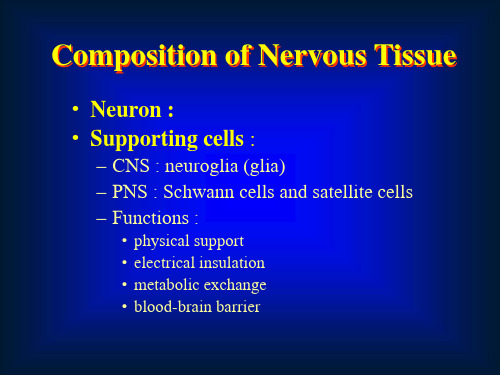
Metabolism of Astrocytes and their Metabolic Cooperation with Neurons
• Glucose • Amino acid metabolism • Enzymes:
• glutamine synthetase(glutamate-glutamine cycle); • glycogen phosphorylatase (glycogen mobilization); • pyruvate carboxylase (anaplerotic synthesis of
BBB
Glia are different from neurons:
1. Neurons have TWO "processes" called axons and dendrites. Glial cells only have ONE.
2. Neurons CAN generate action potentials. Glial cells CANNOT, however, do have a resting potential.
Provide the insulation (myelin) to neurons in the central nervous system.
Satellite Cells Schwann Cells
Physical support to neurons in the peripheral nervous system.
oxaloacetate); • cysteine sulfinate decarboxylase (synthesis of
GFAP抗体
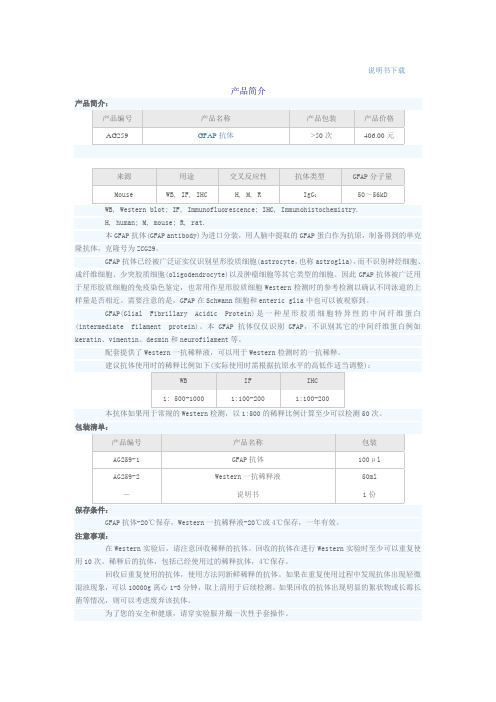
说明书下载产品简介产品简介:产品编号产品名称产品包装产品价格AG259GFAP抗体>50次406.00元来源用途交叉反应性抗体类型GFAP分子量Mouse WB, IF, IHC H, M, R IgG150~56kDWB, Western blot; IF, Immunofluorescence; IHC, Immunohistochemistry.H, human; M, mouse; R, rat.本GFAP抗体(GFAP antibody)为进口分装,用人脑中提取的GFAP蛋白作为抗原,制备得到的单克隆抗体,克隆号为ZCG29。
GFAP抗体已经被广泛证实仅识别星形胶质细胞(astrocyte,也称astroglia),而不识别神经细胞、成纤维细胞、少突胶质细胞(oligodendrocyte)以及肿瘤细胞等其它类型的细胞。
因此GFAP抗体被广泛用于星形胶质细胞的免疫染色鉴定,也常用作星形胶质细胞Western检测时的参考检测以确认不同泳道的上样量是否相近。
需要注意的是,GFAP在Schwann细胞和enteric glia中也可以被观察到。
GFAP(Glial Fibrillary Acidic Protein)是一种星形胶质细胞特异性的中间纤维蛋白(intermediate filament protein)。
本GFAP抗体仅仅识别GFAP,不识别其它的中间纤维蛋白例如keratin、vimentin、desmin和neurofilament等。
配套提供了Western一抗稀释液,可以用于Western检测时的一抗稀释。
建议抗体使用时的稀释比例如下(实际使用时需根据抗原水平的高低作适当调整):WB IF IHC1: 500-1000 1:100-200 1:100-200本抗体如果用于常规的Western检测,以1:500的稀释比例计算至少可以检测50次。
包装清单:产品编号产品名称包装AG259-1 GFAP抗体100μlAG259-2 Western一抗稀释液50ml-说明书1份保存条件:GFAP抗体-20℃保存,Western一抗稀释液-20℃或4℃保存,一年有效。
最新astrocyte(星形胶质细胞)
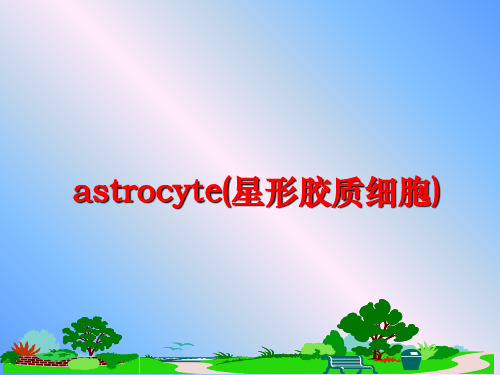
Summary
1 We have reported that tumor necrosis factor (TNF)-α and interleukin (IL)-1β are produced by cultured neurons and mainly by glial cells exposed to unconjugated bilirubin (UCB). The effects of these cytokines are mediated by cell surface receptors through a nuclear factor (NF)-κB-dependent pathway that we have showed to be activated by UCB.
26
25
Hence, cytokine pathways should be taken in consideration when treating UCB-induced neurological dysfunction, emerging as new targets for drug intervention in the prevention of potential brain deficits resulting from neonatal jate that astrocytes exposed to UCB preferentially express TNFR1 rather than TNFR2, at least during the first 24h of treatment, suggesting that TNF- α action in our study model occurs essentially through TNFR1.
神经科学专业英语词汇
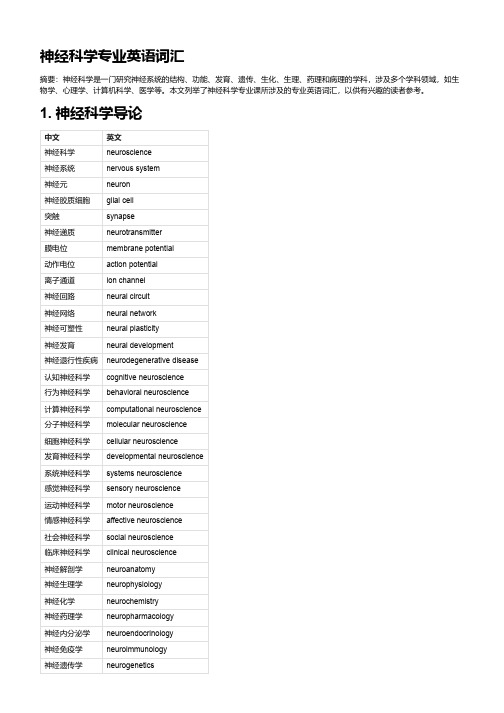
神经科学专业英语词汇摘要:神经科学是一门研究神经系统的结构、功能、发育、遗传、生化、生理、药理和病理的学科,涉及多个学科领域,如生物学、心理学、计算机科学、医学等。
本文列举了神经科学专业课所涉及的专业英语词汇,以供有兴趣的读者参考。
1. 神经科学导论中文英文神经科学neuroscience神经系统nervous system神经元neuron神经胶质细胞glial cell突触synapse神经递质neurotransmitter膜电位membrane potential动作电位action potential离子通道ion channel神经回路neural circuit神经网络neural network神经可塑性neural plasticity神经发育neural development神经退行性疾病neurodegenerative disease认知神经科学cognitive neuroscience行为神经科学behavioral neuroscience计算神经科学computational neuroscience分子神经科学molecular neuroscience细胞神经科学cellular neuroscience发育神经科学developmental neuroscience系统神经科学systems neuroscience感觉神经科学sensory neuroscience运动神经科学motor neuroscience情感神经科学affective neuroscience社会神经科学social neuroscience临床神经科学clinical neuroscience神经解剖学neuroanatomy神经生理学neurophysiology神经化学neurochemistry神经药理学neuropharmacology神经内分泌学neuroendocrinology神经免疫学neuroimmunology神经遗传学neurogenetics2. 神经元和神经胶质细胞中文英文多极神经元multipolar neuron双极神经元bipolar neuron单极神经元(假单极)unipolar neuron (pseudounipolar)轴突(轴索)axon (axis)轴突起始段axon hillock轴突终末axon terminal轴突分支axon collateral轴突鞘axon sheath髓鞘myelin sheath髓鞘间隙(诺氏结)node of Ranvier神经节ganglion神经纤维束nerve fiber神经nerve神经元突起neurite树突dendrite树突棘dendritic spine神经元体(细胞体)soma (cell body)细胞核nucleus细胞质cytoplasm细胞膜cell membrane核糖体ribosome内质网endoplasmic reticulum高尔基体Golgi apparatus线粒体mitochondrion微管microtubule微丝microfilament神经丝(神经原纤维)neurofilament (neurofibril)肌动蛋白actin微管相关蛋白(MAP)microtubule-associated protein (MAP)泰瑞酶(tau蛋白)tau protein (tau)神经纤维缠结(神经原纤维缠结)neurofibrillary tangle (neurofibril tangle)胶质细胞前体(祖细胞)glial progenitor (precursor) cell星形胶质细胞astrocyte沟通型星形胶质细胞protoplasmic astrocyte纤维型星形胶质细胞fibrous astrocyte水肿型星形胶质细胞reactive astrocyte胶质瘢痕glial scar脉络丛上皮细胞choroid plexus epithelial cell中文英文血脑屏障blood-brain barrier胶质限制膜glia limitans脑脊液cerebrospinal fluid微胶质细胞microglia髓鞘形成胶质细胞myelinating glial cell中枢髓鞘形成细胞(少突胶质细胞)central myelinating cell (oligodendrocyte)周围髓鞘形成细胞(雪旺氏细胞)peripheral myelinating cell (Schwann cell)雷迪克氏细胞(卫星细胞)satellite cell (enteric glial cell)神经干细胞neural stem cell神经前体细胞(祖细胞)neural progenitor (precursor) cell神经分化neural differentiation神经迁移neural migration3. 突触和神经递质中文英文突触synapse突触前膜presynaptic membrane突触后膜postsynaptic membrane突触间隙synaptic cleft突触小泡synaptic vesicle突触囊泡synaptic vesicle突触囊泡融合synaptic vesicle fusion突触囊泡回收synaptic vesicle recycling突触囊泡转运synaptic vesicle transport突触囊泡动力学synaptic vesicle dynamics突触递质释放synaptic transmitter release突触递质回收synaptic transmitter uptake突触递质降解synaptic transmitter degradation突触递质受体synaptic transmitter receptor突触递质效应synaptic transmitter effect神经递质neurotransmitter经典神经递质classical neurotransmitter非经典神经递质non-classical neurotransmitter小分子神经递质small molecule neurotransmitter大分子神经递质(神经肽)large molecule neurotransmitter (neuropeptide)乙酰胆碱(ACh)acetylcholine (ACh)乙酰胆碱酯酶(AChE)acetylcholinesterase (AChE)乙酰胆碱受体(AChR)acetylcholine receptor (AChR)胆碱能神经元(ACh神经元)cholinergic neuron (ACh neuron)胆碱能受体cholinergic receptor离子型胆碱能受体(nAChR)ionotropic cholinergic receptor (nAChR)代谢型胆碱能受体(mAChR)metabotropic cholinergic receptor (mAChR)神经肌肉接头neuromuscular junction副交感神经系统parasympathetic nervous system交感神经系统sympathetic nervous system胆碱能神经递质系统cholinergic neurotransmitter system多巴胺(DA)dopamine (DA)多巴胺合成酶(TH)dopamine synthase (TH)多巴胺转运蛋白(DAT)dopamine transporter (DAT)多巴胺受体(DR)dopamine receptor (DR)多巴胺能神经元(DA神经元)dopaminergic neuron (DA neuron)多巴胺能神经递质系统dopaminergic neurotransmitter system基底神经节basal ganglia黑质substantia nigra纹状体striatum前额叶皮层prefrontal cortex奖赏系统reward system去甲肾上腺素(NE)norepinephrine (NE)去甲肾上腺素合成酶(DBH)norepinephrine synthase (DBH)去甲肾上腺素转运蛋白(NET)norepinephrine transporter (NET)去甲肾上腺素受体(AR)adrenergic receptor (AR)去甲肾上腺素能神经元(NE神经元)noradrenergic neuron (NE neuron)去甲肾上腺素能神经递质系统noradrenergic neurotransmitter system蓝斑locus coeruleus脑干brainstem脑血管cerebral blood vessel应激反应stress response血清素(5-HT)serotonin (5-HT)血清素合成酶(TPH)serotonin synthase (TPH)血清素转运蛋白(SERT)serotonin transporter (SERT)血清素受体(5-HTR)serotonin receptor (5-HTR)血清素能神经元(5-HT神经元)serotonergic neuron (5-HT neuron)血清素能神经递质系统serotonergic neurotransmitter system神经管raphe nuclei杏仁核amygdala海马hippocampus松果体pineal gland节律调节circadian rhythm regulation情绪调节emotional regulation记忆形成memory formation抑郁症depression谷氨酸(Glu)glutamate (Glu)谷氨酸合成酶(GAD)glutamate synthase (GAD)谷氨酸转运蛋白(GLT)glutamate transporter (GLT)谷氨酸受体(GluR)glutamate receptor (GluR)谷氨酸能神经元(Glu神经元)glutamatergic neuron (Glu neuron)谷氨酸能神经递质系统glutamatergic neurotransmitter system离子型谷氨酸受体(iGluR)ionotropic glutamate receptor (iGluR)代谢型谷氨酸受体(mGluR)metabotropic glutamate receptor (mGluR) NMDA受体NMDA receptorAMPA受体AMPA receptorKA受体KA receptor长时程增强(LTP)long-term potentiation (LTP)长时程抑制(LTD)long-term depression (LTD)神经元突触可塑性synaptic plasticity学习和记忆learning and memory兴奋性毒性(凋亡)excitotoxicity (apoptosis)脑缺血cerebral ischemia脑损伤brain injury神经退行性疾病neurodegenerative diseaseγ-氨基丁酸(GABA)gamma-aminobutyric acid (GABA)GABA合成酶(GAD)GABA synthase (GAD)GABA转运蛋白(GAT)GABA transporter (GAT)GABA受体(GABAR)GABA receptor (GABAR)GABA能神经元(GABA神经元)GABAergic neuron (GABA neuron)GABA能神经递质系统GABAergic neurotransmitter system离子型GABA受体(GABAA和GABAC)ionotropic GABA receptor (GABAA and GABAC)代谢型GABA受体(GABAB)metabotropic GABA receptor (GABAB)抑制性突触传递inhibitory synaptic transmission抑制性突触后电位(IPSP)inhibitory postsynaptic potential (IPSP)突触抑制synaptic inhibition神经元抑制neuronal inhibition脑内抑制平衡inhibitory balance in the brain神经元兴奋性调节neuronal excitability regulation神经元同步化neuronal synchronization神经振荡neural oscillation4. 神经元膜电位和动作电位中文英文神经元膜电位neuronal membrane potential静息膜电位resting membrane potential膜阻抗membrane impedance膜电容membrane capacitance膜电阻membrane resistance膜导电membrane conductance电流current电压voltage电阻resistance导电conductance电路circuit欧姆定律Ohm's law电池battery电源power source电位差potential difference电容capacitance电荷charge电场electric field离子ion阳离子cation阴离子anion离子浓度ion concentration离子梯度ion gradient离子平衡ion equilibrium离子通道ion channel通透性permeability选择性selectivity电化学梯度electrochemical gradient动力学平衡dynamic equilibrium考克斯-福斯特方程(Nernst方程)Nernst equation (Goldman-Hodgkin-Katz equation)霍奇金-赫胥黎方程(GHK方程)Goldman-Hodgkin-Katz equation (GHK equation)电流-电压关系current-voltage relationship伊凡诺夫-马克斯韦尔方程(IV方程)I-V equation (Ivanov-Maxwell equation)离子通道类型ion channel type电压门控离子通道voltage-gated ion channel配体门控离子通道ligand-gated ion channel机械门控离子通道mechanosensitive ion channel热门控离子通道thermosensitive ion channel光门控离子通道light-gated ion channel离子通道结构ion channel structure离子通道亚基ion channel subunit离子通道孔域ion channel pore domain离子通道电压传感器ion channel voltage sensor离子通道配体结合位点ion channel ligand binding site离子通道状态ion channel state开放状态open state关闭状态closed state不应期refractory period激活activation失活inactivation钠离子通道sodium ion channel钾离子通道potassium ion channel钙离子通道calcium ion channel氯离子通道chloride ion channel动作电位action potential动作电位阈值action potential threshold动作电位上升相action potential rising phase动作电位峰值action potential peak动作电位下降相action potential falling phase动作电位超极化相(余极化相)action potential hyperpolarization phase (afterhyperpolarization phase)动作电位传播action potential propagation电缆理论cable theory电缆方程cable equation空间常数space constant时间常数time constant电流源current source电流汇current sink电流注入current injection电流刺激current stimulation电极electrode钳制技术clamp technique电压钳制voltage clamp电流钳制current clamp轴突初始段axon initial segment节间区internode region髓鞘myelin髓鞘形成myelination髓鞘退化demyelination髓鞘病变myelinopathy多发性硬化症(MS)multiple sclerosis (MS)传导速度conduction velocity盐跃式传导saltatory conduction节段性传导segmental conduction节段性抑制segmental inhibition节段性增强segmental facilitation节段性可塑性segmental plasticity5. 神经元突触传递和突触可塑性中文英文突触传递synaptic transmission突触前神经元presynaptic neuron突触后神经元postsynaptic neuron突触前释放presynaptic release突触后反应postsynaptic response突触后电位(PSP)postsynaptic potential (PSP)兴奋性突触后电位(EPSP)excitatory postsynaptic potential (EPSP)抑制性突触后电位(IPSP)inhibitory postsynaptic potential (IPSP)突触整合synaptic integration空间整合spatial summation时间整合temporal summation神经元输出neuronal output神经元输入-输出函数neuronal input-output function神经元输入阻抗neuronal input impedance神经元输出阻抗neuronal output impedance突触可塑性synaptic plasticity突触强度synaptic strength突触增强synaptic potentiation突触抑制synaptic depression短时程可塑性short-term plasticity长时程可塑性long-term plasticity短时程突触增强(STP)short-term potentiation (STP)短时程突触抑制(STD)short-term depression (STD)长时程突触增强(LTP)long-term potentiation (LTP)长时程突触抑制(LTD)long-term depression (LTD)突触前可塑性presynaptic plasticity突触后可塑性postsynaptic plasticityHebb学习规则Hebbian learning rule反Hebb学习规则anti-Hebbian learning rule突触修剪synaptic pruning6. 神经元网络和神经编码神经元网络neuronal network神经元群neuronal population神经元集合neuronal ensemble神经元回路neuronal circuit神经元模块neuronal module神经元连接neuronal connection突触连接synaptic connection电隙连接(电耦合)gap junction (electrical coupling)化学突触(化学耦合)chemical synapse (chemical coupling)突触权重synaptic weight突触矩阵synaptic matrix连接拓扑connection topology连接图connectivity graph连接概率connection probability连接密度connection density神经元活动neuronal activity神经元放电neuronal firing神经元放电频率neuronal firing rate神经元放电模式neuronal firing pattern神经元放电相位neuronal firing phase神经元放电序列neuronal firing sequence神经元放电同步neuronal firing synchronization神经元放电相关性neuronal firing correlation神经元放电耦合neuronal firing coupling神经元放电编码neuronal firing coding神经编码neural coding速率编码rate coding时序编码temporal coding相位编码phase coding空间编码spatial coding模式编码pattern coding群体编码population coding稀疏编码sparse coding分布式编码distributed coding局部化编码localized coding神经解码neural decoding逆向重建inverse reconstruction信息论information theory信息量information content信息熵information entropy互信息mutual information信噪比signal-to-noise ratio噪声抑制noise suppression信号增强signal enhancement信号处理signal processing7. 神经元网络模型和神经元网络仿真中文英文神经元网络模型neuronal network model神经元模型neuron model突触模型synapse model电路模型circuit model网络拓扑模型network topology model网络动力学模型network dynamics model网络功能模型network function model生物实际性(生物合理性)biological realism (biological plausibility)计算效率computational efficiency数学分析mathematical analysis数值仿真numerical simulation仿真软件simulation software仿真平台simulation platform仿真语言simulation language仿真脚本simulation script神经元网络仿真neuronal network simulation仿真结果simulation result仿真分析simulation analysis仿真验证simulation validation仿真优化simulation optimization仿真可视化simulation visualization仿真数据simulation data仿真参数simulation parameter仿真变量simulation variable仿真常量simulation constant仿真函数simulation function仿真对象simulation object仿真事件simulation eventHodgkin-Huxley模型(HH模型)Hodgkin-Huxley model (HH model) FitzHugh-Nagumo模型(FHN模型)FitzHugh-Nagumo model (FHN model)莱克-范德波尔模型(LIF模型)Leaky integrate-and-fire model (LIF model)伊兹克维奇模型(Izhikevich模型)Izhikevich model (Izhikevich model)中文英文NEURON软件NEURON software GENESIS软件GENESIS software NEST软件NEST software Brian软件Brian software PyNN软件PyNN software NEURON语言NEURON language HOC语言HOC language Python语言Python language MATLAB语言MATLAB language C语言 C languageC++语言C++ language Java语言Java languageR语言R language。
卡达尔健康肾上腺素产品说明书
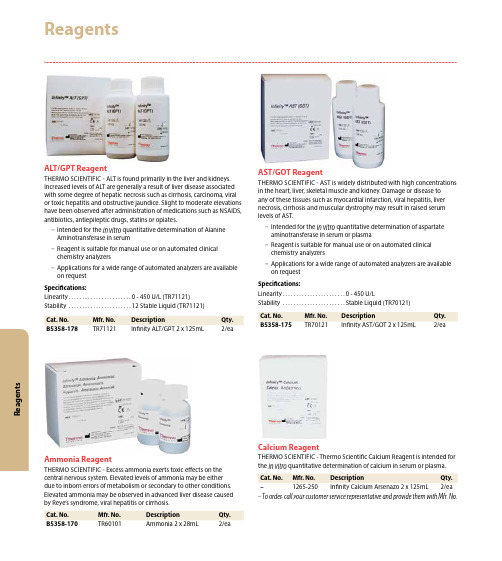
R e a g e n t sALT/GPT ReagentTHERMO SCIEnTIFIC - AlT is found primarily in the liver and kidneys . Increased levels of AlT are generally a result of liver disease associated with some degree of hepatic necrosis such as cirrhosis, carcinoma, viral or toxic hepatitis and obstructive jaundice . Slight to moderate elevations have been observed after administration of medications such as nSAIDS, antibiotics, antiepileptic drugs, statins or opiates .ūIntended for the in vitro quantitative determination of Alanine Aminotransferase in serumūReagent is suitable for manual use or on automated clinical chemistry analyzersūApplications for a wide range of automated analyzers are available on requestSpecifications:linearity . . . . . . . . . . . . . . . . . . . . . . .0 - 450 U/l (TR71121)Stability . . . . . . . . . . . . . . . . . . . . . . .12 Stable liquid (TR71121)Cat. No.Mfr. No.DescriptionQty.B5358-178TR71121Infinity AlT/GPT 2 x 125ml2/eaAmmonia ReagentTHERMO SCIEnTIFIC - Excess ammonia exerts toxic effects on the central nervous system . Elevated levels of ammonia may be either due to inborn errors of metabolism or secondary to other conditions . Elevated ammonia may be observed in advanced liver disease caused by Reye’s syndrome, viral hepatitis or cirrhosis .Cat. No.Mfr. No.DescriptionQty.B5358-170TR60101Ammonia 2 x 28ml2/eaAST/GOT ReagentTHERMO SCIEnTIFIC - AST is widely distributed with high concentrations in the heart, liver, skeletal muscle and kidney . Damage or disease to any of these tissues such as myocardial infarction, viral hepatitis, liver necrosis, cirrhosis and muscular dystrophy may result in raised serum levels of AST .ūIntended for the in vitro quantitative determination of aspartate aminotransferase in serum or plasmaūReagent is suitable for manual use or on automated clinical chemistry analyzersūApplications for a wide range of automated analyzers are available on requestSpecifications:linearity . . . . . . . . . . . . . . . . . . . . . . .0 - 450 U/lStability . . . . . . . . . . . . . . . . . . . . . . .Stable liquid (TR70121)Cat. No.Mfr. No.DescriptionQty.B5358-175TR70121Infinity AST/GOT 2 x 125ml2/eaCalcium ReagentTHERMO SCIEnTIFIC - Thermo Scientific Calcium Reagent is intended for the in vitro quantitative determination of calcium in serum or plasma .Cat. No.Mfr. No.DescriptionQty.–1265-250Infinity Calcium Arsenazo 2 x 125ml2/ea– To order, call your customer service representative and provide them with Mfr. No.。
glia细胞的分类 -回复
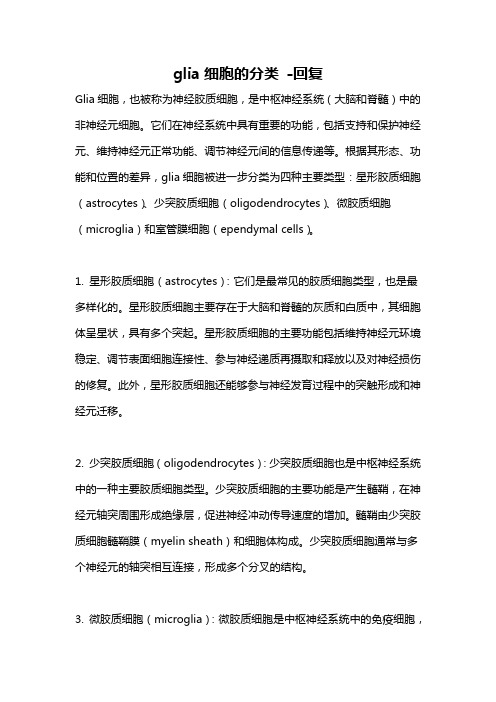
glia细胞的分类-回复Glia细胞,也被称为神经胶质细胞,是中枢神经系统(大脑和脊髓)中的非神经元细胞。
它们在神经系统中具有重要的功能,包括支持和保护神经元、维持神经元正常功能、调节神经元间的信息传递等。
根据其形态、功能和位置的差异,glia细胞被进一步分类为四种主要类型:星形胶质细胞(astrocytes)、少突胶质细胞(oligodendrocytes)、微胶质细胞(microglia)和室管膜细胞(ependymal cells)。
1. 星形胶质细胞(astrocytes):它们是最常见的胶质细胞类型,也是最多样化的。
星形胶质细胞主要存在于大脑和脊髓的灰质和白质中,其细胞体呈星状,具有多个突起。
星形胶质细胞的主要功能包括维持神经元环境稳定、调节表面细胞连接性、参与神经递质再摄取和释放以及对神经损伤的修复。
此外,星形胶质细胞还能够参与神经发育过程中的突触形成和神经元迁移。
2. 少突胶质细胞(oligodendrocytes):少突胶质细胞也是中枢神经系统中的一种主要胶质细胞类型。
少突胶质细胞的主要功能是产生髓鞘,在神经元轴突周围形成绝缘层,促进神经冲动传导速度的增加。
髓鞘由少突胶质细胞髓鞘膜(myelin sheath)和细胞体构成。
少突胶质细胞通常与多个神经元的轴突相互连接,形成多个分叉的结构。
3. 微胶质细胞(microglia):微胶质细胞是中枢神经系统中的免疫细胞,起到清除神经系统中的病原体、细胞垃圾和细胞凋亡产物的作用。
当神经系统发生病理性改变(如炎症、感染)时,微胶质细胞会被激活并释放趋化因子,引起免疫细胞的迁移和炎症反应。
因此,微胶质细胞在神经系统中具有重要的免疫调节功能。
4. 室管膜细胞(ependymal cells):室管膜细胞主要存在于脑室和脊髓中央管腔表面。
它们形成了形状各异的上皮细胞层,具有微绒毛状突起。
室管膜细胞的主要功能包括产生脑脊液、维持脑脊液的稳定性、促进脑脊液的循环和运输。
神经系统(英1)
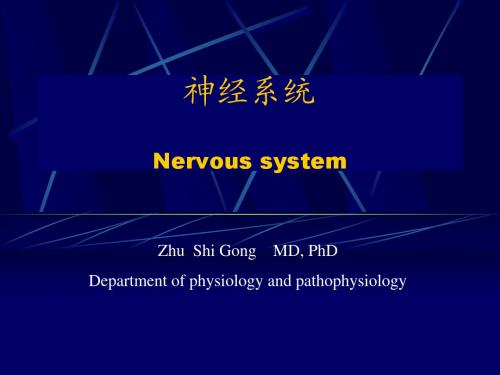
20-80nm
20nm
7.5nm 7.5nm
(2).神经元之间其它联系方式
A.Gap junction(缝隙连接 (电突触): 缝隙连接)( 缝隙连接 电突触) 两个神经元膜紧密连接部位。间隙比突触间 隙小得多。无突触小泡。接触部位电阻较低。其 传递方式不是化学传递,而是电传递,也称为电 突触。传递快,几乎无潜伏期。如小脑篮状细胞。 B. Nonsynaptic chemical transmission(非突触性 非突触性 化学传递): 化学传递 在轴突末梢的分支上进行。分支上有许多典 张体,内含装有递质小泡。递质释放后,通过弥 散到达附近的效应细胞发挥作用。如单胺类神经 纤维末梢。
Function of neuroglia
支持作用:为neuron提供支架。如Astrocyte 运输营养物质:如Astrocyte有些长突起末端膨大(周 足,end foot),终止于毛细胞血管壁上及细胞元上, 起到运输营养物质作用。还能产生神经营养性因子。 分隔与绝缘作用: CNS中各区域及neuron之间分隔, 形 成髓鞘(oligodendrocyte). 修复与再生作用: Astrocyte吞噬溃变碎片,并填充形 成胶质瘢痕(glial scar), 增殖的胶质细胞又称为反应性 胶质细胞(reactive glia),可释放神经营养因子,刺激 神经细胞生长,有利损伤的再生和修复。
Axon----soma
(Axosomatic)
Axon---dentrite (Axodendritic) Axon---axon (Axoaxonal)
Dentrite---dentrite (dendrodendritic)
Structure of synapes
经典突触结构包括: Presynaptic membrane Postsynaptic membrane Synaptic vesicles(突触小泡) chemical substance (neurotransmitter)
glia细胞的分类 -回复
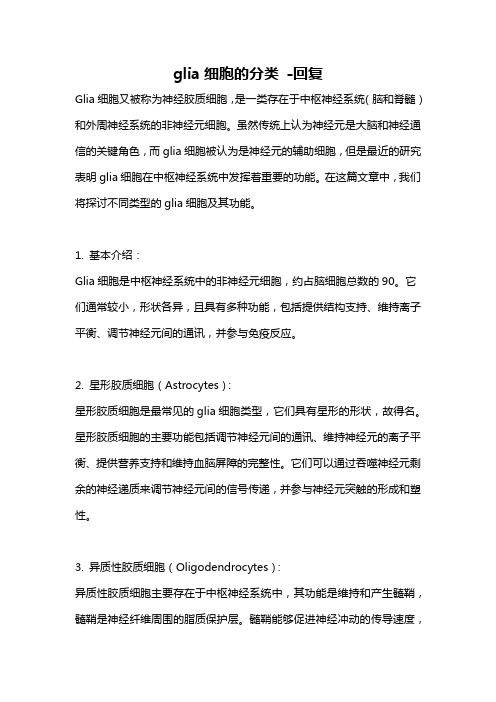
glia细胞的分类-回复Glia细胞又被称为神经胶质细胞,是一类存在于中枢神经系统(脑和脊髓)和外周神经系统的非神经元细胞。
虽然传统上认为神经元是大脑和神经通信的关键角色,而glia细胞被认为是神经元的辅助细胞,但是最近的研究表明glia细胞在中枢神经系统中发挥着重要的功能。
在这篇文章中,我们将探讨不同类型的glia细胞及其功能。
1. 基本介绍:Glia细胞是中枢神经系统中的非神经元细胞,约占脑细胞总数的90。
它们通常较小,形状各异,且具有多种功能,包括提供结构支持、维持离子平衡、调节神经元间的通讯,并参与免疫反应。
2. 星形胶质细胞(Astrocytes):星形胶质细胞是最常见的glia细胞类型,它们具有星形的形状,故得名。
星形胶质细胞的主要功能包括调节神经元间的通讯、维持神经元的离子平衡、提供营养支持和维持血脑屏障的完整性。
它们可以通过吞噬神经元剩余的神经递质来调节神经元间的信号传递,并参与神经元突触的形成和塑性。
3. 异质性胶质细胞(Oligodendrocytes):异质性胶质细胞主要存在于中枢神经系统中,其功能是维持和产生髓鞘,髓鞘是神经纤维周围的脂质保护层。
髓鞘能够促进神经冲动的传导速度,因此,异质性胶质细胞起到了重要的神经元保护和功能加快的作用。
4. 微胶质细胞(Microglia):微胶质细胞是中枢神经系统中的免疫细胞,它们是最小的glia细胞类型,形状呈细长的圆柱状。
微胶质细胞具有清除脑组织中的病原体、死亡细胞和细胞垃圾的功能,类似于宏噬细胞。
此外,微胶质细胞还能产生和释放免疫调节因子,参与炎症反应。
5. 忍氏胶质细胞(Ependymal Cells):忍氏胶质细胞主要分布在脑室和中央导水管内,它们具有上皮细胞的特征,有绒毛突起。
忍氏胶质细胞的主要功能是分泌脑脊液和参与细胞外液平衡的调节。
6. 盧氏胶质细胞(Bergmann Glia):盧氏胶质细胞存在于小脑中,其主要功能是促进神经元的迁移和正常的组织结构形成。
阿绍夫细胞名词解释

阿绍夫细胞名词解释一、引言阿绍夫细胞(Astrocyte)是中枢神经系统(Central Nervous System,CNS)中的一种神经胶质细胞,其主要功能是提供结构支持、调节离子平衡、参与能量代谢以及调控神经元之间的通讯。
本文将对阿绍夫细胞的结构、功能和生理学作用进行详细解释。
二、结构特点阿绍夫细胞具有以下几个主要结构特点:1.星状形态:阿绍夫细胞在组织中呈现出星状或分枝状的形态,这种形态使得它们能够紧密与其他神经元和血管相连。
2.足突:阿绍夫细胞具有多个足突,这些足突可以覆盖大片区域,并与其他神经元和血管发生联系。
这些足突不仅提供了机械支持,还通过足突间的连接与其他神经元进行信号传递。
3.星形纤毛:在阿绍夫细胞的表面上可以观察到一些星形纤毛,这些纤毛有助于增加细胞表面积,提供更多的接触点。
三、功能作用阿绍夫细胞在中枢神经系统中发挥着重要的功能作用:1.结构支持:阿绍夫细胞通过与血管和其他神经元的连接,为中枢神经系统提供结构支持。
它们形成了一个网状结构,稳定了神经组织的排列和空间布局。
2.离子平衡调节:阿绍夫细胞可以调节神经元周围的离子平衡,特别是钠、钾和氯离子的浓度。
通过吸收或释放这些离子,阿绍夫细胞可以控制神经元的兴奋性。
3.能量代谢参与:阿绍夫细胞参与神经元的能量代谢过程。
它们可以吸收葡萄糖并将其转化为能量供给周围的神经元使用。
4.养护修复:当中枢神经系统受到损伤时,阿绍夫细胞会发生活化,并释放出一系列生长因子和细胞因子,促进神经元的再生和修复。
5.神经递质清除:阿绍夫细胞还具有清除神经递质的功能,通过摄取周围的神经递质,阻止它们在突触间隙中过度积累。
四、与其他细胞的关系阿绍夫细胞与其他神经元和胶质细胞之间存在密切的相互作用:1.神经元:阿绍夫细胞与神经元之间通过足突和突触结构相连。
它们可以接收神经元释放的信号,并对其进行调节和传导。
2.星形胶质细胞:阿绍夫细胞是星形胶质细胞(Astroglia)家族中的一员,与其他星形胶质细胞共同构成了中枢神经系统的主要组成部分。
走,去太空采矿!
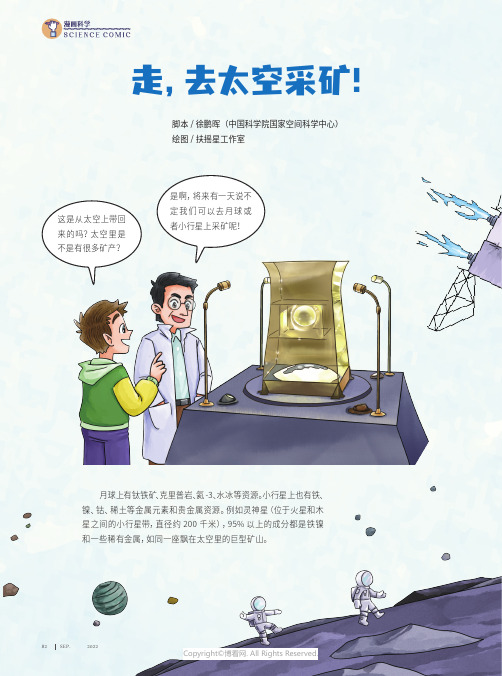
脚本/徐鹏晖(中国科学院国家空间科学中心)绘图/扶摇星工作室
走,去太空采矿!
这是从太空上带回
来的吗?太空里是
不是有很多矿产?是啊,将来有一天说不定我们可以去月球或者小行星上采矿呢!月球上有钛铁矿、克里普岩、氦-3、水冰等资源。
小行星上也有铁、镍、钴、稀土等金属元素和贵金属资源。
例如灵神星(位于火星和木星之间的小行星带,直径约200千米),95%以上的成分都是铁镍和一些稀有金属,如同一座飘在太空里的巨型矿山。
202282SEP.Copyright ©博看网. All Rights Reserved.
被绿色荧光标记的细菌正在“啃食”岩石使用细菌之一:鞘氨醇单胞菌目前,科学家还在探索如何对太空资源进行高精度测绘与勘探,如何在太空失重环境下进
行钻探与采集等。
相信随着科学技术的进步,去太空采矿将不再是遥不可及的梦想!(责任编辑 / 陈琛 美术编辑 / 韦英章)
我们可以在月球和火星上建基地,将收集的资源就地提炼、利用;用无人航天器
将小行星拖到地球轨道附近,利用某些能“吃”岩石的细菌帮我们在天体上采矿。
细菌怎么“吃”岩石啊?其实那是一种化学分解过程。
这些岩石在与细菌一起培养21天后,其中的金
属元素会被浸出,岩石也变成海绵样疏松多孔的状
态,如同被“吃”了一般。
Copyright ©博看网. All Rights Reserved.。
tyrax材料化学名称

tyrax材料化学名称【实用版】目录1.引言:介绍泰拉克斯材料化学的背景和重要性2.泰拉克斯材料化学的定义和分类3.泰拉克斯材料化学的特性和应用4.泰拉克斯材料化学的发展前景和挑战5.结论:总结泰拉克斯材料化学的价值和意义正文一、引言随着科技的不断发展,新材料已经成为了各个领域中不可或缺的元素。
其中,泰拉克斯材料化学作为一种新兴的材料化学,逐渐受到科研界和工业界的关注。
本文将详细介绍泰拉克斯材料化学的名称及其相关内容。
二、泰拉克斯材料化学的定义和分类泰拉克斯材料化学,又称太拉化学,来源于英文 Tellurium(泰拉)和 Chemistry(化学)的组合。
它是研究泰拉元素(Te)与其他元素在化学反应中的性质、结构、制备和应用的一门学科。
泰拉克斯材料化学可以分为以下几个主要类别:1.无机泰拉化学:研究泰拉元素与非金属元素形成的无机化合物,如氧化物、硫化物、卤化物等。
2.有机泰拉化学:研究泰拉元素与碳原子形成的有机化合物,如泰拉烃、泰拉醇、泰拉酮等。
3.物理泰拉化学:研究泰拉元素在不同条件下的物理性质,如熔点、沸点、密度等。
4.生物泰拉化学:研究生物体内泰拉元素的分布、代谢、功能等生物学性质。
三、泰拉克斯材料化学的特性和应用泰拉克斯材料化学具有以下特性:1.半导体特性:泰拉元素具有半导体特性,可用于制备高性能的电子器件。
2.光电特性:泰拉元素的光电特性使其在光电器件中有广泛的应用。
3.催化特性:泰拉元素具有一定的催化活性,可用于催化化学反应。
4.环保特性:泰拉元素可用于制备环保材料,如降解塑料等。
泰拉克斯材料化学在多个领域具有广泛的应用,如电子工业、光电产业、能源领域、环境保护等。
随着研究的深入,泰拉克斯材料化学的应用范围将不断扩大。
四、泰拉克斯材料化学的发展前景和挑战泰拉克斯材料化学作为一种新兴的材料化学,具有巨大的发展潜力。
然而,目前对其研究仍面临一些挑战,如:1.理论研究不足:对泰拉元素的理论研究还不够深入,需要加强基础研究。
- 1、下载文档前请自行甄别文档内容的完整性,平台不提供额外的编辑、内容补充、找答案等附加服务。
- 2、"仅部分预览"的文档,不可在线预览部分如存在完整性等问题,可反馈申请退款(可完整预览的文档不适用该条件!)。
- 3、如文档侵犯您的权益,请联系客服反馈,我们会尽快为您处理(人工客服工作时间:9:00-18:30)。
Astrocyte Calcium Waves:What They Are and What They DoELIANA SCEMES1*AND CHRISTIAN GIAUME2*1Department of Neuroscience,Albert Einstein College of Medicine,Bronx,New Y ork2College de France,Paris,FranceKEY WORDSglial cells;gliotransmitters;ATP;gap junctions;connexins ABSTRACTSeveral lines of evidence indicate that the elaborated cal-cium signals and the occurrence of calcium waves in astro-cytes provide these cells with a specific form of excitability. The identification of the cellular and molecular steps in-volved in the triggering and transmission of Ca21waves between astrocytes resulted in the identification of two path-ways mediating this form of intercellular communication. One of them involves the direct communication between the cytosols of two adjoining cells through gap junction channels, while the other depends upon the release of‘‘gliotrans-mitters’’that activates membrane receptors on neighboring cells.In this review we summarize evidence in favor of these two mechanisms of Ca21wave transmission and we discuss that they may not be mutually exclusive,but are likely to work in conjunction to coordinate the activity of a group of cells.To address a key question regarding the functional consequences following the passage of a Ca21wave,we list, in this review,some of the potential intracellular targets of these Ca21transients in astrocytes,and discuss the func-tional consequences of the activation of these targets for the interactions that astrocytes maintain with themselves and with other cellular partners,including those at the glial/vas-culature interface and at perisynaptic sites where astrocytic processes tightly interact with neurons.V C2006Wiley-Liss,Inc. CALCIUM SIGNALS AS A SPECIFIC MODE OF EXCITABILITY AND TRANSMISSION INASTROCYTES:HISTORICAL PERSPECTIVE Thefindings that astrocytes express a variety of ion channels and membrane receptors,which enable them to respond on a millisecond time scale to neuronal activity with changes in membrane potential and/or increases in intracellular Ca21levels(Barres et al.,1990;MacVicar and Tse,1988;Marrero et al.,1989;McCarthy and Salm, 1991;Salm and MacCarthy,1990;Usowic et al.,1989)was thefirst step in the gliafield leading to the hypothesis that these cells could play a role in CNS information pro-cessing.It was based on these early reports that Cornell-Bell et al.(1990)and Charles et al.(1991)first reported that astrocytes were not only able to respond to external stimulation with increases in intracellular calcium eleva-tions but,most importantly,they were be able to transmit these calcium signals to adjacent non-stimulated astro-cytes,as intercellular Ca21waves(ICWs).The presence of such phenomenon of propagating waves of calcium lead to the proposition that‘‘networks of astrocytes constitute an extraneuronal pathway for rapid long-distance signal transmission within the CNS.’’Moreover,these authors (Cornell-Bell et al.,1990)proposed that‘‘if Ca21activity in the network of astrocytes constituted another form of intercellular communication,such signaling should have a physiological relevance influencing neuronal activity, and thus being bi-directional.’’Work by several independ-ent groups showed that indeed there is a reciprocal com-munication between neurons and astrocytes.Hippocam-pal neuronal activity was shown to trigger calcium waves in astrocyte networks(Dani et al.,1992)and astrocyte cal-cium waves were shown to modulate neuronal activity (Dani et al.,1992;Kang et al.,1998;Nedergaard,1994; Parpura et al.,1994;Parri et al.,2001).The mode by which astrocyte calcium signals affect synaptic transmis-sion was then shown to be dependent on regulated exocy-tosis of stored glutamate,ATP,and D-serine(Bezzi et al., 2004;Coco et al.,2003;Mothet et al.,2005;Parpura et al., 1994;Pascual et al.,2005).Consequently,the pre-and post-synaptic components of neuronal transmission gained a new partner,the perisynaptic glia,forming together what was initially termed the‘‘tripartite-synapse’’(Araque et al.,1998a,b).Because these studies indicated that the electrically silent astrocytes were active participants of CNS information processing,it became plausible to con-sider that astrocytes are nevertheless‘‘excitable’’cells, with Ca21fluctuations being the signal by which they re-spond,integrate,and convey information.Although with the limitation of the studies performed in culture,pioneering experiments(Cornell-Bell et al., 1990)generated insightful ideas that,followed by experi-mental evidence,brought a new perspective to the role of glial cells in CNS function.Of note is their report on the spatial and temporal pattern changes that occur after 100l M glutamate application.Following the initial Ca21 elevation induced by receptor activation,oscillatory Ca21 Grant sponsor:National Institutes of Health;Grant number:RO1-NS41023to ES;Grant sponsor:INSERM.*Correspondence to:Eliana Scemes,Department of Neuroscience,Kennedy Center, Room No.203,Albert Einstein College of Medicine,1410Pelham Parkway,Bronx,NY 10461,USA.E-mail:scemes@;Christian Giaume,INSERM-U587,Col-lege de France,11Place Marcelin Berthelot,Paris75005,France.E-mail:christian.giaume@college-de-franceReceived21March2006;Accepted23May2006DOI10.1002/glia.20374Published online26September2006in Wiley InterScience(www.interscience. ).GLIA54:716–725(2006)V C2006Wiley-Liss,Inc.fluctuations preceded the intercellular spread of Ca 21.This oscillatory behavior persisted for long periods of time (5–30min)with variable frequencies (10–110mHz).A direct correlation between glutamate concentration and frequency of oscillations was observed:at low concentra-tions (below 1l M)intracellular Ca 21transients were asynchronous and localized,whereas at higher concentra-tions (10–100l M)intercellular Ca 21waves propagated over long distances.This indicated that small fluctuations in intracellular Ca 21could be integrated to generate a global intracellular response,which could be transmitted throughout the astrocytic network.Recent development of in vivo imaging,using two-photon microscopy,has pro-vided evidence for coordinated astrocyte Ca 21activity in the neocortex of rats (Hirase et al.,2004).Although many of the questions that were raised when Ca 21waves were first described have been answered,many are still debated and others have been generated.This review is not intended to revisit the concept and evi-dence that culminated in this new line of investigation,for which several recent reviews are available (Charles and Giaume,2002;Nedergaard et al.,2003;Scemes,2000).Instead,this review will focus on the characteristics and consequences of intercellular Ca 21signaling in astrocytes and their relevance to CNS function.Because‘‘gliotransmitter’’released from astrocytes (the mechan-isms by which this release is accomplished are discussed in the other reviews),besides affecting synaptic transmis-sion and brain microcirculation,is also likely to serve as an autocrine signal,we will consider in this review some important aspects of this feedback mechanism for the transmission of Ca 21waves between astrocytes.CA 21WAVES IN VITRO AND IN VIVO:WHENAND WHERE DO THEY OCCUR?A calcium wave is defined as a localized increase in cy-tosolic Ca 21that is followed by a succession of similar events in a wave-like fashion.These Ca 21waves can be restricted to one cell (intracellular)or transmitted to neigh-boring cells (intercellular)(Fig.1A).The basic steps that lead to intracellular Ca 21waves in astrocytes usually involve the activation of G-protein-coupled receptors,activation of phospholipase C,and the production of IP 3,which following IP 3R activation leads to Ca 21release from the endoplasmic reticulum (ER)(Golo-vina and Blaustein,2000;Scemes,2000;Sheppard et al.,1997).These intracellular Ca 21signals are spatially and temporally complex events involving the recruitmentofFig.1.Intercellular Ca 21waves and their intracellular targets.(A )The transmission of intercellular Ca 21signals between astrocytes is illu-strated in the sequential images obtained from Fluo-3-AM loaded spinal cord astrocytes.Mechanical stimulation (arrow)of a single astrocyte in culture induces intracellular Ca 21elevation (displayed as an increase in fluorescence intensity)in the stimulated cells,which is then followed by Ca 21increases in neighboring astrocytes.Images were acquired with an Orca-ER CCD camara attached to Nikon TE2000inverted microscope equipped with a 310objective,using Metafluor software.Bar:50l m.(B )The diagram illustrates the major intracellular targets of cytosolic Ca 21fluctuations in astrocytes.Elevation of intracellular Ca 21levels isshown to affect (arrows)several plasma membrane proteins (symbols refer from left to right to metabotropic receptors,K 1(Ca 21)channels,Na 1/Ca 21exchanger,and Ca 21-ATPase),as well as intracellular ones.The inositol-trisphosphate receptors (IP 3R)are located at the endoplas-mic reticulum (ER)where Ca 21exert a cooperative action.Rises in [Ca 21]i also target several cytoskeleton elements (cytosk),enzymes (E),and vesicles involved on the release of ‘‘gliotransmitters’’.Finally,Ca 21and the Ca 21liberating second messenger IP 3permeate gap junction channels and then act on similar intracellular targets in neighboring coupled cells.717INTERCELLULAR CALCIUM WAVESGLIA DOI 10.1002/gliaelementary Ca21release sites(Ca21puffs:Parker and Yao,1994),which then propagate throughout the cell by an amplification mechanism.This amplification involves four components,two of which depend on positive and two on negative feedback mechanisms provided by released Ca21.These feedback mechanisms are:(a)the activation of nearby IP3Rs due to the co-agonistic action of Ca21on these receptors(Bezprovanny and Ehrlich,1995; Finch et al.,1991;Yao et al.,1995),(b)the additional gen-eration of IP3through the Ca21-dependent activation of PLC(Berridge,1993;Venance et al.,1997),(c)the buffer-ing power of mitochondria,attenuating the excess Ca21 levels at IP3R microdomains that otherwise would reduce the sensitivity of these receptors to IP3(Boitier et al., 1999;Simpson et al.,1998),and(d)the presence of endog-enous low affinity Ca21buffers(calcium binding proteins) that limit the diffusion of Ca21ions within single astro-cytes(Wang et al.,1997).Once triggered,intracellular Ca21waves can be trans-mitted to neighboring cells as ICWs.Regardless of the mechanism by which these waves may travel(see details that follow),the mechanism that triggers Ca21transients in adjacent astrocytes relies on IP3production and subse-quent release of Ca21from the ER,as summarized above for intracellular Ca21waves.Therefore,the extent to which these intercellular Ca21waves can travel are gov-erned by the effective diffusion properties of the Ca21 mobilizing signaling molecules within and between cells. ICW spread between astrocytes derived from cell cul-ture,brain slice,and whole retina preparations has been observed following pharmacological,electrical,and me-chanical stimulation(for review see Boitier et al.,1999; Charles,1998;Charles and Giaume,2002;Giaume and Venance,1998;Newman,2004;Scemes,2000;Simpson et al.,1998).Although there are some differences regard-ing the distance and shape of ICWs generated by each type of stimulation,once they are initiated,the velocities by which they travel between astrocytes are fairly simi-lar,independent of the mode of stimulation and type of preparation.For instance,pharmacological,mechanical, and electrical stimulation of rat retina induced ICWs that traveled at a mean speed of23l m/sec(Newman and Zahs,1997);pharmacologically and mechanically-induced intercellular Ca21waves between cultured astrocytes travel at a mean velocity of18l m/sec(for reviews see Giaume and Venance,1998;Scemes,2000),and in the electrically stimulated brain slice preparations,the mean Ca21wave velocity is15l m/sec(Dani et al.,1992;Hass et al.,2005;Schipke et al.,2002).In contrast to the veloc-ity of Ca21wave spread,the extent to which ICW travels is highly variable,even when comparing a single type of stimulation among different types of preparations.For example,differences in the number of cells participating in ICW transmission following mechanical stimulation were observed among cultures of astrocytes prepared from distinct brain regions;ICWs spread to twice as many cor-tical and hippocampal astrocytes as between astrocytes from the hypothalamus and brain stem(Blomstrand et al.,1999).Similarly,mechanically induced Ca21waves between cultured telencephalic astrocytes spread radially to an area of450l m2comprising about400cells while waves traveling between cultured diencephalic astrocytes spread unevenly to an area of130l m2recruiting about 100cells(Peters et al.,2005).Regardless of the notorious differences between culture and in situ conditions(e.g.,morphology of astrocytes,size of the extracellular space),some generalizations can be made with respect to the properties of ICWs.Based on the studies reported above,it is likely that some of the charac-teristics of Ca21waves are governed by the intrinsic prop-erties of the astrocytic Ca21signaling toolkit(Berridge et al.,2000a,b)—G-coupled membrane receptors,Ca21 mobilizing second messengers,ER,Ca21buffering mole-cules and intracellular organelles—that once fully activated to produce an intracellular Ca21wave can be transmitted to an adjacent astrocyte,with a velocity that is independ-ent of the type of preparation used,i.e.,independent of the morphological differences.Moreover,even considering that the extent of ICW spread is vastly larger in culture conditions than in brain slices,most likely due to the re-stricted extracellular space in the latter,the studies per-formed in cultured astrocytes from different brain regions described above suggest that the distance of transmission of ICW is likely related to heterogeneous populations of astrocytes.Differences in type and number of membrane receptors and gap junction channels,main components of astrocyte intercellular Ca21signaling(see following),are likely responsible for defining boundaries of communicat-ing networks.Although these in vitro studies indicate that astrocytes can to some variable extent and degree transmit ICWs,the magnitude of the stimuli necessary to trigger this form of Ca21signaling is usually considerably higher than would be expected to occur under physiological situation.Thus,the existence and relevance of this form of astrocytic communication for CNS function may be questioned at least in normal,physiological situations.In whole-mount retinas,flickering light stimulation indu-ces Ca21transients,but not intercellular Ca21waves in Mueller cells of the inner plexiform layer;however,in the presence of adenosine,lightflashes enhance Mueller cell responses and induce ICW spread between these glial cells(Newman,2005).This study suggested that ICW propagation between Mueller cells may occur under non-physiological conditions,such as under hypoxic condi-tions where adenosine levels are significantly increased (Ribelayga and Mangel,2005).However,it should be noted that adenosine levels in retinas alsofluctuate in a circadian and light-dependent manner(Ribelayga and Mangel,2005)and therefore,ICW spread between Muel-ler cells may occur under physiological conditions.Simi-larly,using two-photon laser scanning microscopy to monitor in vivo Ca21dynamics in astrocytes from superfi-cial cortical layer of rat brains,Hirase et al.(2004) recorded low frequency Ca21transients in single astro-cytes that could be spread,although within a very limited range,between few neighboring cells with a low but non-zero level of coordination;the degree of such coordinated Ca21activity between neighboring astrocytes was found to be positively correlated with neuronal discharge,as718SCEMES AND GIAUME GLIA DOI10.1002/gliaobserved following blockade of neuronal GABA A recep-tors with bicuculline.Thus,this study provided evidence that enhanced but not seizure-related neuronal activity influences intercellular glia communication in the intact brain.In view of what is known so far about ICWs in astro-cytes,it is likely that this form of Ca21signal transmis-sion play important role under pathological but not under physiological conditions.With the exception of early CNS developmental stages,when spontaneous Ca21waves are frequently observed and implicated in the generation,dif-ferentiation,and migration of neural cells(Feller et al., 1996;Gu and Spitzer,1995;Kumada and Komuro,2004; Scemes et al.,2003;Weissman et al.,2004;Wong et al., 1995),spontaneous ICWs are rarely seen in the mature CNS,even following physiological stimulation.PATHWAYS FOR INTERCELLULARTRANSMISSION OF CA2+SIGNALSIN ASTROCYTESThere are two possible pathways by which Ca21signals can be transmitted between cells;one involves the trans-fer of Ca21mobilizing second messengers directly from the cytosol of one cell to that of an adjacent one through gap junction intercellular channels and the other involves the‘‘de novo’’generation of such messengers in neighbor-ing cells through the activation of membrane receptors due to extracellular diffusion of agonists.These two path-ways are not mutually exclusive but are likely to work in conjunction to provide coordinated activity within groups of cells.Gap junction mediated transmission of ICWs was the first pathway identified in astrocytes(Finkbeiner,1992). In this study it was shown that neither the direction nor the velocity of glutamate-induced intercellular Ca21 waves were affected by rapid superfusion and that two gap junction channel blockers impaired Ca21wave spread between astrocytes without affecting this spread within cells.Thisfinding together with several others performed in different systems(Blomstrand et al.,1999;Charles et al.,1991,1992;Enkvist and McCarthy,1992;Guan et al.,1997;Leybaert et al.,1998;Nedergaard,1994;Saez et al.,1989;Scemes et al.,1998;Venance et al.,1995)pro-vided a strong basis supporting the view that gap junction channels played a crucial role in the transmission of Ca21 signals between astrocytes.It should be mentioned,how-ever,that some of these early experiments performed using compounds that block gap junction channels may have not allowed the selective discrimination between gap junction-dependent and-independent pathways for ICW transmission.For instance,several gap junction channel/ hemichannel blockers(heptanol,octanol,carbenoxolone,flufenamic acid,and mefloquine)have been recently reported to prevent ATP-dependent amplification of ICWs under low divalent cation solutions by acting on P2X7 receptors(Suadicani et al.,2006).Evidence for the participation of an extracellular path-way for the spread of intercellular Ca21waves in astro-cytes,first described in the non-coupled mast cells(Osip-chuk and Cahalan,1992),was provided by Enkvist and McCarthy(1992),showing that Ca21waves could cross cell bare areas in confluent cultures of cerebral astrocytes. Later,Hassinger et al.(1996)showed that electrically-induced Ca21waves in cultured astrocytes were able to cross cell-free areas up to120l m and travel with similar velocity between confluent cells and through the acellular lanes;moreover,the extent and direction of waves travel-ing in confluent cultures were shown to be affected by superfusion(Hassinger et al.,1996).It was then demon-strated that ATP was the extracellular molecule released by stimulated astrocytes and that this messenger also mediated the acellular transmission of Ca21waves(Guthrie et al.,1999).Suchfindings provided an explanation for the results showing that Ca21waves were not totally abol-ished in conditions where coupling was reduced,as in the case of astrocytes derived from Cx43-null mice(Naus et al.,1997;Scemes et al.,1998),or astrocytes treated with oleomide and anandamide,a condition that totally prevented dye-and electrical-coupling(Guan et al.,1997), and why blocking purinergic receptors with the P2R an-tagonist suramin totally prevented this spread(Guan et al.,1997;Zanotti and Charles,1997).As mentioned above,calcium signals can be transmitted through the diffusion of an extracellular molecule acting on membrane receptors.Except for striatal astrocytes,which display a prominent Ca21response to glutamate(Cornell-Bell et al.,1990;Finkbeiner,1992),astrocytes from other brain regions,including the cortex(Guthrie et al.,1999), retina(Newman and Zahs,1997)and spinal cord(Scemes et al.,2000;Scemes,unpublished observations),are more responsive to ATP than to glutamate.Astrocytes in situ and in vitro express,at different levels,several ionotropic and metabotropic P2purinergic receptors,some of which have been implicated in the transmission of calcium sig-nals(Fumagalli et al.,2003).Among the metabotropic P2Y receptors,the P2Y1R and P2Y2R subtypes are likely those predominantly expressed in astrocytes(Ho et al., 1995;Idestrup and Salter,1998;Zhu and Kimelberg, 2001,2004).Although both of these G-coupled P2Rs gen-erate PLC and IP3upon stimulation and thus contribute to generating Ca21elevations,they differ with regard to their sensitivity and selectivity for nucleotides;with the exception of ATP that is an agonist(in the micromolar range)at both receptors,the purine diphosphate nucleo-tide ADP is a potent agonist(in the nanomolar range) at the P2Y1R while the pyrimidine triphosphate nucleo-tide UTP stimulates(in the micromolar range)P2Y2R but not P2Y1R(Burnstock and Knight,2004).Given the presence of ectonucleotidases at the cell surface that readily hydrolyze ATP to form ADP before the full hydro-lysis into adenosine(Wink et al.,2006),signaling through P2Y1R activation is likely to predominate.Using a P2R-null astrocytoma(1321N1)cell line to selectively express P2YR,Gallagher and Salter(2003) and Suadicani et al.(2004)reported that both P2Y1R and P2Y2R were equally efficient to sustain Ca21wave spread and that,however,the properties(velocity,extent,and shape)of these waves differed.Although differences in719INTERCELLULAR CALCIUM WAVESGLIA DOI10.1002/gliasensitivity of these two P2receptor subtypes to ATP can in part explain why ICWs traveling between P2Y1R and P2Y2R differ(Gallagher et al.,2003),it is also likely that gap junction channels contribute to modulating the veloc-ity,distance,and the shape of these extracellular gener-ated waves.For instance,by overexpressing the Cx43in this poorly coupled astrocytoma cell line stably expres-sing P2Y1R and P2Y2R subtypes,a40%decrease in the rate of ICW transmission was observed(Suadicani et al., 2004).Moreover,overexpression of Cx43in P2Y1R and P2Y4R-cells caused dramatic changes in the shape and distance of ICW spread;the non-wave shape,saltatory behavior of Ca21waves traveling amongst poorly coupled P2Y1R expressing cells assumed a more homogeneous, wave-shaped form in better coupled P2Y1R astrocytoma cells,while the limited spread of Ca21signals provided by P2Y4R,was practically abolished by increasing gap junctional communication(Suadicani et al.,2004).These studies illustrate that the properties of Ca21sig-nal transmission not only depend on the(sub)-type of membrane receptors but also on the degree of gap junc-tion mediated intercellular coupling.If,for example,an agonist(at its maximal effective concentration at a parti-cular receptor)is not sufficient to generate appropriate quantities of Ca21mobilizing second messengers(e.g., IP3)to sustain long distance Ca21signal transmission, the increase in the effective volume of the intracellular compartment provided by the gap junction channels will certainly dissipate the gradient to levels below threshold and the wave will be terminated(Giaume and Venance, 1998;Suadicani et al.,2004).Alternatively,gap junction channels could recruit non-responsive cells into a net-work of cells expressing receptors that more efficiently generate second messengers(Venance et al.,1998).Thus, as initially proposed(Hassinger et al.,1996),both gap junction dependent and independent pathways partici-pate in the transmission of Ca21signals between astro-cytes;however,the relative contribution of each of these pathways is likely to depend upon developmental,re-gional,and physiological states.Accordingly,it has been recently shown in acute brain slices that depending on the brain regions(cortex versus hippocampus and corpus callosum),the pathway mediating the transmission of Ca21signals in brain slices is different(Haas et al., 2006).Another example illustrating that Ca21waves can utilize different routes when traveling between glial cells was provided in whole mounts of mouse retina,where astrocyte-to-astrocyte Ca21waves are mainly mediated by the diffusion of second messengers through gap junc-tion channels,whereas astrocyte-to-Mueller cells are ba-sically dependent on the diffusion of ATP through the extracellular space(Newman,2001,2003,2004).REGENERATIVE VS NON-REGENERATIVE INTERCELLULAR CA21WAVE TRANSMISSION For both gap junction-dependent and-independent pathways discussed above,the basic law governing Ca21 signal transmission is provided by diffusion equations,and therefore,diffusional parameters are expected to play a significant role limiting the extent to which these signals propagate.However,regenerative models for Ca21trans-mission have been proposed.These models are derived from evidence obtained from several independent groups indicating that,upon stimulation,astrocytes were able to release ATP and glutamate(Ballerini et al.,1996;Kimel-berg et al.,1990,1995;Parpura et al.,1994,1995;Queiroz et al.,1997,1999).The release of these Ca21-mobilizing ‘‘gliotransmitters’’can potentially feedback on the astrocy-tic population,in an autocrine fashion,thus amplifying the extent to which these Ca21signals are transmitted (Stout et al.,2002;Suadicani et al.,2006).In the studies performed by Hassinger et al.(1996)and Guthrie et al. (1999)showing that astrocyte Ca21waves depended upon the release of the extracellular messenger ATP,the authors also proposed a mechanistic model by which ATP released from the stimulated cells would activate P2R receptors,which would then lead to mobilization of intra-cellular Ca21in the neighboring cell that in turn would be followed by the release of ATP from this neighboring cell. This succession of events would then occur sequentially along the ICW path.Although recent evidence supports the hypothesis that ATP induces ATP release from astro-cytes(Anderson et al.,2004),this regenerative ATP release model,however,does not explain why Ca21waves travel within defined limits.By taking advantage of the dominant role of gap junctions in the propagation of cal-cium waves in rat striatal astrocytes,Giaume and Venance(1998)proposed that limiting factors linked to in-tracellular calcium signaling(PLC activity,calcium buf-fering,filling and re-filling of internal stores)contribute to setting a threshold in cells at the edge of the ICW that resulted in stopping the propagation.However,this pro-cess of ICW spread through gap junctions is not totally passive but requires a regenerative process dependent upon calcium activation of PLC g to produce new IP3in the receiving cells.This regenerative but limited intercel-lular signaling was then tested by a mathematical approach that reproduced the propagation properties of ICWs(Hofer et al.,2002).As a counter-proposal for such regenerative model of Ca21wave transmission,Nedergaard’s group(Arcuino et al.,2002;Cotrina et al.,1998,2000)suggested a non-re-generative model based on a point source of ATP release, such that ATP released from a single cell would diffuse and stimulate a limited number of nearby cells.Evidence in favor of such a non-regenerative model of Ca21wave transmission is their observation that only cells located at the epicenter of a spontaneously generated Ca21wave were permeable to molecules(propidium iodide;562Da) large enough to allow the release of ATP(Arcuino et al., 2002)and that the distance traveled by waves crossing cell-free areas was the same as those traveling between cells(Arcuino et al.,2004).This point source release mech-anism of initiation of ICWs from the stimulated cell,to-gether with P2R activation and gap junction-mediated dif-fusion of IP3,have been incorporated into a recent mathe-matical model of ICW transmission in astrocytes(Iacobas et al.,2006).720SCEMES AND GIAUME GLIA DOI10.1002/glia。