Soil organic carbon loss under different slope gradients in loess hilly region
土壤有机碳 激发效应
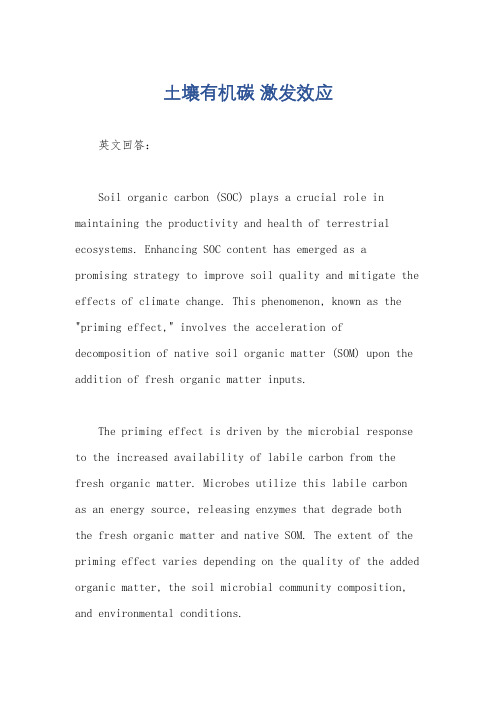
土壤有机碳激发效应英文回答:Soil organic carbon (SOC) plays a crucial role in maintaining the productivity and health of terrestrial ecosystems. Enhancing SOC content has emerged as a promising strategy to improve soil quality and mitigate the effects of climate change. This phenomenon, known as the "priming effect," involves the acceleration of decomposition of native soil organic matter (SOM) upon the addition of fresh organic matter inputs.The priming effect is driven by the microbial response to the increased availability of labile carbon from the fresh organic matter. Microbes utilize this labile carbon as an energy source, releasing enzymes that degrade both the fresh organic matter and native SOM. The extent of the priming effect varies depending on the quality of the added organic matter, the soil microbial community composition, and environmental conditions.Several mechanisms have been proposed to explain the priming effect. One theory suggests that the addition of fresh organic matter stimulates the growth of microbial populations, leading to increased enzyme production and decomposition of both the fresh and native SOM. Another mechanism involves the selective utilization of labile carbon by microbes, leaving behind more recalcitrant compounds that are more resistant to decomposition. This process can result in the accumulation of recalcitrant organic matter, which can have long-term effects on soil carbon dynamics.The priming effect can have both positive and negative implications for soil health and ecosystem functioning. On the one hand, it can accelerate the release of nutrients from SOM, making them available for plant uptake. This increased nutrient availability can boost plant growth and productivity. On the other hand, the priming effect can also lead to the loss of stable SOC, which is an important component of soil carbon storage and a major contributor to the global carbon cycle.Managing the priming effect is crucial for sustainable soil management practices. One approach involves the use of organic matter amendments that are high in labile carbonand low in recalcitrant compounds. This can help tominimize the loss of stable SOC while still stimulating microbial activity and nutrient release. Additionally, maintaining a diverse soil microbial community can promote the balanced decomposition of organic matter and reduce the risk of excessive priming.中文回答:土壤有机碳(SOC)在维持陆地生态系统的生产力和健康方面发挥着至关重要的作用。
土壤学双语试题
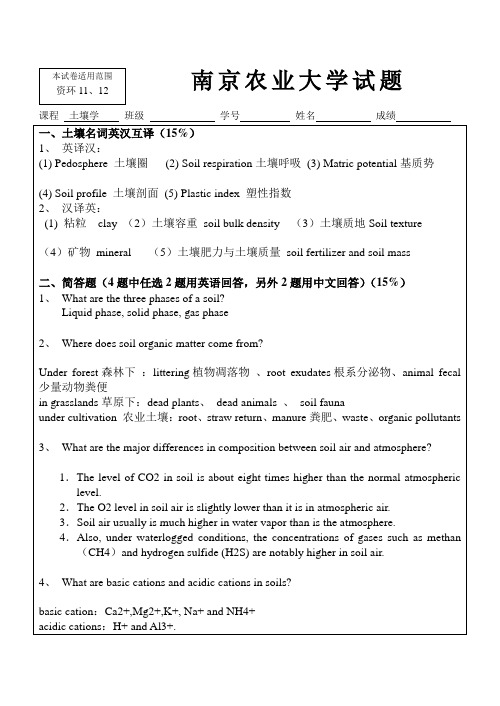
9.Sand has less nutrients for plants than smaller particles
4、影响土壤氧化还原的因素为:①土壤通气性②微生物活动③易分解有机质的含量④植物根系的代谢作用⑤土壤pH。
5、土壤热量的来源主要有:①Solar radiation②biologicalheat③heat insidetheea土壤有何肥力特点? 土壤质地的改良措施有哪些?
四、填空题(20%)
1、土壤容重值的用途为①计算土壤孔隙度②计算工程土方量③估算各种土壤成分储量④计算土壤储水量与灌水(排水)定额。
2、目前国内外流行的质地分类制主要为:①International②USDA③Kachinsky④Chinese四种。
3、土壤水分含量的表示方法主要有:①Themass water content②Thevolumn water content③relative water content④Soil water-storage capacity四种。
8、适宜作物生长发育的耕层总孔度为(D)。
A、8%-10%;B、15%-20%;C、50%-56%;D、60%以上
9、土壤中热容量最大的是(B)。水最大,气体最小
A、气体;B、水;C、矿物质;D、有机质
10、旱地土壤的氧化还原电位(Eh)一般为(D)。
A、-200 mv以下; B、-200-100mv;C、100mv左右;D、400-700mv
5.Some sands in soil will be brown, yellow, or red because of Fe and/or Al oxide coatings.
土壤碳的固持 英语

土壤碳的固持英语Soil Carbon sequestration: An Essential Process for Sustainable Agriculture and Climate Change Mitigation.Soil carbon sequestration, also known as soil carbon storage or soil carbon fixation, is a critical process in maintaining the health and productivity of soil ecosystems. It involves the stabilization and accumulation of carbon in soil organic matter, which can occur through various biological, chemical, and physical processes. This process is crucial for sustainable agriculture, climate change mitigation, and the overall well-being of the planet.The importance of soil carbon sequestration cannot be overstated. Soils are the largest terrestrial carbon pool, storing more carbon than the atmosphere and all plant biomass combined. This carbon is stored in the form of organic matter, which is composed of dead and decaying plant and animal remains, as well as living microorganisms. When carbon is sequestered in soil organic matter, it iseffectively removed from the atmosphere, thus reducing the concentration of greenhouse gases and mitigating theimpacts of climate change.There are several key processes involved in soil carbon sequestration. One of the most important is photosynthesis, which occurs in plants and converts carbon dioxide into organic matter. When plants die and decay, their carbon-rich remains are incorporated into soil organic matter, contributing to carbon sequestration. Additionally, soil microorganisms play a crucial role in decomposing organic matter and releasing carbon dioxide, which can then be re-fixed by plants through photosynthesis.The management of agricultural systems cansignificantly impact soil carbon sequestration. For example, the use of organic matter in soil amendments, such as compost or manure, can increase the amount of carbon insoil organic matter. Conservation agriculture practices, such as no-till farming or crop rotation, can also enhance soil carbon sequestration by reducing soil erosion and increasing soil organic matter content.The benefits of soil carbon sequestration extend beyond climate change mitigation. Healthy soils are essential for sustainable agriculture, providing a foundation for crop growth and yield. Soil organic matter improves soil structure, water retention, and nutrient cycling, all of which are crucial for crop productivity. By sequestering carbon in soil organic matter, farmers can enhance the fertility and resilience of their soils, leading to improved crop yields and reduced dependence on external inputs.In addition to its agricultural benefits, soil carbon sequestration can also contribute to the achievement of global climate goals. The Intergovernmental Panel on Climate Change (IPCC) has identified soil carbon sequestration as one of the most effective and cost-efficient strategies for reducing greenhouse gas emissions and mitigating climate change. By increasing soil carbon sequestration, we can not only reduce the amount of carbon dioxide in the atmosphere but also enhance the resilience and productivity of our agricultural systems.In conclusion, soil carbon sequestration is a crucial process for sustainable agriculture, climate change mitigation, and the overall well-being of the planet. It involves the stabilization and accumulation of carbon in soil organic matter, which can be enhanced through various agricultural management practices. By sequestering carbon in soil organic matter, we can reduce greenhouse gas emissions, improve soil health and fertility, andcontribute to the achievement of global climate goals. As we face the challenges of climate change and sustainable development, soil carbon sequestration remains an essential component of our efforts to protect our planet and ensure a sustainable future for all.。
Soil organic carbon as functions of slope aspects and soil depths
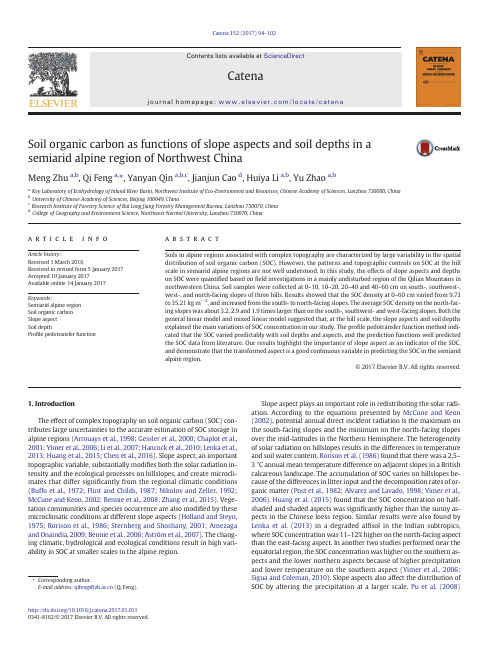
Soil organic carbon as functions of slope aspects and soil depths in a semiarid alpine region of Northwest ChinaMeng Zhu a ,b ,Qi Feng a ,⁎,Yanyan Qin a ,b ,c ,Jianjun Cao d ,Huiya Li a ,b ,Yu Zhao a ,baKey Laboratory of Ecohydrology of Inland River Basin,Northwest Institute of Eco-Environment and Resources,Chinese Academy of Sciences,Lanzhou 730000,China bUniversity of Chinese Academy of Sciences,Beijing 100049,China cResearch Institute of Forestry Science of Bai Long Jiang Forestry Management Bureau,Lanzhou 730070,China dCollege of Geography and Environment Science,Northwest Normal University,Lanzhou 730070,Chinaa b s t r a c ta r t i c l e i n f o Article history:Received 1March 2016Received in revised form 5January 2017Accepted 10January 2017Available online 14January 2017Soils in alpine regions associated with complex topography are characterized by large variability in the spatial distribution of soil organic carbon (SOC).However,the patterns and topographic controls on SOC at the hill scale in semiarid alpine regions are not well understood.In this study,the effects of slope aspects and depths on SOC were quanti fied based on field investigations in a mainly undisturbed region of the Qilian Mountains in northwestern China.Soil samples were collected at 0–10,10–20,20–40and 40–60cm on south-,southwest-,west-,and north-facing slopes of three hills.Results showed that the SOC density at 0–60cm varied from 9.73to 35.21kg m −2,and increased from the south-to north-facing slopes.The average SOC density on the north-fac-ing slopes was about 3.2,2.9and 1.9times larger than on the south-,southwest-and west-facing slopes.Both the general linear model and mixed linear model suggested that,at the hill scale,the slope aspects and soil depths explained the main variations of SOC concentration in our study.The pro file pedotransfer function method indi-cated that the SOC varied predictably with soil depths and aspects,and the prediction functions well predicted the SOC data from literature.Our results highlight the importance of slope aspect as an indicator of the SOC,and demonstrate that the transformed aspect is a good continuous variable in predicting the SOC in the semiarid alpine region.©2017Elsevier B.V.All rights reserved.Keywords:Semiarid alpine region Soil organic carbon Slope aspect Soil depthPro file pedotransfer function1.IntroductionThe effect of complex topography on soil organic carbon (SOC)con-tributes large uncertainties to the accurate estimation of SOC storage in alpine regions (Arrouays et al.,1998;Gessler et al.,2000;Chaplot et al.,2001;Yimer et al.,2006;Li et al.,2007;Hancock et al.,2010;Lenka et al.,2013;Huang et al.,2015;Chen et al.,2016).Slope aspect,an important topographic variable,substantially modi fies both the solar radiation in-tensity and the ecological processes on hillslopes,and create microcli-mates that differ signi ficantly from the regional climatic conditions (Buffo et al.,1972;Flint and Childs,1987;Nikolov and Zeller,1992;McCune and Keon,2002;Bennie et al.,2008;Zhang et al.,2015).Vege-tation communities and species occurrence are also modi fied by these microclimatic conditions at different slope aspects (Holland and Steyn,1975;Rorison et al.,1986;Sternberg and Shoshany,2001;Amezaga and Onaindia,2009;Bennie et al.,2006;Aström et al.,2007).The chang-ing climatic,hydrological and ecological conditions result in high vari-ability in SOC at smaller scales in the alpine region.Slope aspect plays an important role in redistributing the solar radi-ation.According to the equations presented by McCune and Keon (2002),potential annual direct incident radiation is the maximum on the south-facing slopes and the minimum on the north-facing slopes over the mid-latitudes in the Northern Hemisphere.The heterogeneity of solar radiation on hillslopes results in the differences in temperature and soil water content.Rorison et al.(1986)found that there was a 2.5–3°C annual mean temperature difference on adjacent slopes in a British calcareous landscape.The accumulation of SOC varies on hillslopes be-cause of the differences in litter input and the decomposition rates of or-ganic matter (Post et al.,1982;Alvarez and Lavado,1998;Yimer et al.,2006).Huang et al.(2015)found that the SOC concentration on half-shaded and shaded aspects was signi ficantly higher than the sunny as-pects in the Chinese loess region.Similar results were also found by Lenka et al.(2013)in a degraded al fisol in the Indian subtropics,where SOC concentration was 11–12%higher on the north-facing aspect than the east-facing aspect.In another two studies performed near the equatorial region,the SOC concentration was higher on the southern as-pects and the lower northern aspects because of higher precipitation and lower temperature on the southern aspect (Yimer et al.,2006;Sigua and Coleman,2010).Slope aspects also affect the distribution of SOC by altering the precipitation at a larger scale.Pu et al.(2008)Catena 152(2017)94–102⁎Corresponding author.E-mail address:qifeng@ (Q.Feng)./10.1016/j.catena.2017.01.0110341-8162/©2017Elsevier B.V.All rightsreserved.Contents lists available at ScienceDirectCatenaj ou r n a l h o m e pa ge :ww w.e l s e v i e r.c o m /l oc a t e /c a t e n afound that the SOC concentration was higher on the windward (east)compared to the leeward (west)slopes in the northern Hengduan Mountains region in southwest China,attributing this distribution pat-tern to the higher precipitation (1735mm)on the windward slopes and lower on the leeward slopes (640mm).Soil depth is an important variable in modeling the vertical distribu-tion pattern of SOC and investigating organic carbon storage at a region-al scale.The vertical distribution pattern of SOC enables us to estimate the organic carbon storage in the subsoil from the topsoil data,and to quantify and map the SOC for different soil depths (Mestdagh et al.,2004;Yang et al.,2007).Generally,the relations between SOC and depths can be expressed by many mathematical functions (exponential,logarithmic,power and quadratic).Among these functions,the expo-nential function was most widely employed (Minasny et al.,2006).In semiarid alpine regions,soil water availability is a main environ-mental control involved in shaping the ecosystem productivity because of the relatively low annual precipitation and strong potential transpira-tion.Topography,which exerts a signi ficant in fluence on the spatial var-iability of soil water content (Li et al.,2015;Gao et al.,2015),may signi ficantly affect the accumulation of organic carbon in semiarid al-pine soils.However,the distribution pattern of SOC at the hillslope scale in semiarid alpine region is less understood,especially in high al-titude regions.This is mainly because of their limited accessibility.Moreover,although the effect of slope aspects on SOC is widely recog-nized,few studies have been conducted to examine the quantitative re-lation of SOC with slope aspects and soil depths (Lenka et al.,2013;Huang et al.,2015;Chen et al.,2016).Therefore,the main goals of this study are (1)to investigate the distribution patterns of SOC along the as-pect and depth gradients,(2)to determine the contributions of slope as-pects and depths to the overall variation of SOC at the hill scale,and (3)to construct the SOC prediction functions with slope aspects and soil depths as independent variables.2.Materials and methods 2.1.Study areaThe study was carried out in the Xishui Forest Zone (100°03′–100°23′E,38°23′–38°48′N)of the Qilian Mountains National Forest Re-serve,which lies in the north edge of Qinghai –Tibet Plateau,Northwest-ern China.The Xishui Forest Zone covers an area of 7239.8km 2with elevation ranging from 2400to 4000m,and is characterized by a semi-arid climate with an annual average temperature of 0.7°C and annual average precipitation of 435mm (Chang et al.,2013).The landscape in the study area is forest-steppe,which represents the main landscape type in the middle Qilian Mountains (Lu et al.,2001).Vegetationpatterns are closely related to the slope aspect,and represent a mosaic of grasslands and forests (Chen et al.,2016).At our sampling sites,grass-lands occupy mainly south-,southwest-,and west-facing slopes with the dominant species including Agropyron cristatum ,Carex crebra ,Stipa capillata Linn,and Kobresia humilis .Forests,dominated by Qinghai spruce (Picea crassifolia ),are mainly distributed on northwest-and north-facing slopes (Table 1).The soils,according to the taxonomic clas-si fication system,are mainly argic-ustic semi-luvisols on north-facing slopes and calcaric-ustic pedocal on other aspects.In the Picea crassifolia forest,there was a clear gravel composition,mainly of calcareous rock,below 60cm.2.2.Experimental designIn this study,we selected three sites in total (Hills A,B,and C)as the replicates with similar landscape type (forest-steppe),elevation (about 2950m),and slope (33°)in the middle Qilian Mountains (Fig.1,Table 1).The relative elevations of the three hills were b 100m,which enabled us to control the effect of elevations on the SOC and to focus on the var-iation of SOC along the aspect gradients.On each hill,we examined tran-sects from the south-(SFS,azimuth angle of 180°),southwest-(SWFS,225°),west-(WFS,270°)to north-facing slope (NFS,360°).Along each transect,three sampling positions (1to 3)were selected from the shoulderslope to footslope position (Fig.1c).Each sampling position contained three plots,and the size of each plot was (5×5)m 2on SFS,SWFS,and WFS (mainly occupied by grasslands),and 10×10m 2on NFS (forests).Within each plot,three soil pro files were excavated (after removing the surface litter layer),and soil samples of 5.00cm in diameter and 5.05cm in length were collected by the core ring method (100cm 3core volume)at 5,15,30,and 50cm,and were oven dried at 105°C for 24h to obtain the dry weight for calculating the bulk density (Blake and Hartge,1986).Soil samples for chemical analyses were col-lected next to each soil pro file using a soil auger (3.5cm in diameter)at 0–10,10–20,20–40,and 40–60cm.Soil samples (three replicates)from each plot were pooled to give a composite sample from each of the four depth intervals.In total,324soil pro files were excavated and 432composite soil samples were collected for SOC concentration anal-ysis in this study.2.3.Soil analysesIn the laboratory,soil samples were air-dried and passed through a 2mm sieve to remove the gravel and roots.SOC concentration was de-termined by wet oxidation with dichromate according to the Walkley –Black method (Nelson and Sommers,1982).Table 1Geographical and vegetative characteristics of the sampled transects on the three hills.HillTransectLength (m)Slope (°)Elevation of sampling position (m)Vegetation typesCover (%)Dominant species123ASFS 5030291229052897grassland 30Agropyron cristatum,Kobresia capillifolia SWFS 7233290328902874grassland 45Agropyron cristatum,Carex crebra WFS 12537290228812849grassland 65Kobresia humilis,Carex crebra NFS 9331290128852864forest 70Picea crassifoliaBSFS 9128301229832964grassland 40Agropyron cristatum,Potentilla bifurca L.SWFS 13834300729792950grassland 60Carex crebra,Potentilla bifurca L.WFS 15736299629502938grassland 70Kobresia humilis,Potentilla bifurca L.NFS 7833300729902974forest 75Picea crassifoliaCSFS 11830296229402928grassland 35Agropyron cristatum,Stipa przewalskyi Roshev.SWFS 16133295829222886grassland 50Carex crebra,Agropyron cristatum WFS 17935295028992866grassland 60Kobresia humilis,Poa annua L.NFS7733296429482939forest75Picea crassifoliaNote:SFS,SWFS,WFS,and NFS are south-,southwest-,west-,and north-facing slopes,respectively.95M.Zhu et al./Catena 152(2017)94–1022.4.Data analysesThe SOC density of each soil pro file was calculated using Eq.(1)as follows:SOCD ¼∑nk ¼1C k D k B k 1−G k ðÞ=100ð1Þwhere SOCD is the SOC density (kg m −2),n is the number of layers di-vided in each soil pro file,and C k ,D k ,B k ,and G k represent the SOC con-centration (g kg −1),the thickness (cm),the bulk density (g cm −3),and the fractional percentage (%)of gravel larger than 2mm in diameter of layer k ,respectively.2.5.Statistical analysesTo investigate the contribution of slope aspects,soil depths and ele-vations to the overall variation of SOC on the three hills,we employed both general linear model (GLM)and mixed linear model (MLM).As the GLM is a linear statistical model,we transformed the aspects and depths with the cosine and logarithmic functions,respectively,and used the transformed aspects and depths as well as the untransformed elevations as the independent variable.In the MLM analysis,we select-ed the aspects,depths,and elevations as fixed-effects parameters,and the hill as a random-effects parameter.The GLM and MLM analyses were performed employing the GLM and MIXED procedures,respec-tively,in SAS 9.2(SAS Institute,Inc.,Cary,NC,USA).2.6.The pro file pedotransfer functionThe pro file pedotransfer function was applied to obtain the empiri-cal equations for predicting the SOC concentration and the density on each hill (Zinn et al.,2005).To describe the vertical distribution of SOC concentration at each as-pect,we applied Eq.(2)as follows:SOC concentration ¼a −b ln h ð2Þwhere h is soil depth (cm),and is included in calculations as the mid-point of sampling layer (e.g.5cm for 0–10cm),and a and b are coef fi-cients estimated by nonlinear regression.The variation of coef ficients a ,and b in Eq.(2)by slope aspect was described by Eq.(3)as follows:a ¼c 1e d 1cos A ðÞb ¼c 2e d 2cos A ðÞð3Þwhere a and b are the coef ficients in Eq.(2),A is azimuth angle of slope aspect (°),and c 1,d 1,c 2,and d 2are coef ficients estimated by nonlinear regression.We substituted Eq.(3)for coef ficients a and b in Eq.(2),and the SOC concentration at different aspects and depths was described by Eq.(4)as follows:SOC concentration ¼c 1e d 1cos A ðÞ−c 2e d 2cos A ðÞln hð4ÞTo quantify the effects of depths on SOC density,we applied the con-tinuous SOC density method (Yang et al.,2007).This method is based on continuous decreases in the SOC density with depth,and differs from the method in Eq.(1),which obtains the SOC density through summing up the densities of all soil layers divided in a soil pro file.The continuous SOC density method enabled us to estimate the SOC density at any given depth (Yang et al.,2007).Therefore,SOC density at depth h can be cal-culated as follows:SOCD h ¼C h B h 1−G k ðÞ=100ð5Þwhere SOCD h (kg m −2cm −1)is SOC density at depth h (cm),and C h ,B h ,and G h are the SOC concentration (g kg −1),the bulk density (g cm −3),and the fractional percentage (%)of gravel larger than 2mm in diameter at depth h ,respectively.Based on the pro file pedotransfer function,we first regressed the re-lation between SOCD h and azimuth angle of aspects,as follows:SOCD h ¼ae b cos A ðÞð6Þwhere a and b are coef ficients estimated by nonlinear regression.The variation of coef ficients a and b in Eq.(6)by depth was de-scribed by Eq.(7)as follows:a ¼c 1þd 1e e 1h b ¼c 2þd 2e e 2hð7Þwhere a and b are coef ficients in Eq.(6),and c 1,d 1,e 1,c 2,d 2,and e 2are coef ficients estimated by nonlinearregression.Fig.1.Location of study area and distribution of sampling plots on hillslopes.(a)Tridimensional surface map of study area,(b)images of Hills A,B and C,(c)3m contour map of Hill A indicating the distribution of soil pro files.The sampling method on Hills B and C is the same as Hill A.The SFS,SWFS,WFS,and NFS represent south-,southwest-,west-,and north-facing slope,respectively.96M.Zhu et al./Catena 152(2017)94–102Thus,the SOC density at0–h was obtained by calculating SOCD h for 0–h,as follows:SOCD¼∫hc1þd1e e1he c2þd2e e2hðÞcos AðÞdhð8Þ3.Results3.1.Distribution patterns of SOCThe SOC concentration in our sampling positions varied from24.10 to112.94,18.61to81.99,12.73to65.01,and8.59to53.08g kg−1at 0–10,10–20,20–40,and40–60cm,respectively(Table1).The SOC den-sity at0–60cm varied from9.73to35.21kg m−2.For grasslands on the SFS,SWFS and WFS,and forests on the NFS,the average SOC density at 0–60cm were13.33and33.64kg m−2,respectively.The results also showed that the SOC concentration increased from the SFS to NFS at each soil depths(Table2).The average topsoil(0–10cm)organic carbon concentration on the NFS(108.44±19.89g kg−1)was larger than on the SFS(25.10±5.70g kg−1), SWFS(28.92±4.75g kg−1),and WFS(54.09±14.01g kg−1)by factors of4.3,3.8,and2.0,respectively,and similar factors between aspects were also obtained at10–20,20–40,and40–60cm.The SOC concentra-tion showed a significantly decreasing trend with soil depth at all sam-pling positions,and the SOC concentration at0–10cm was about2to3 times larger than that at40–60cm.Along the transect from higher to lower elevations,the SOC concentration tended to be larger at the lower positions(except on the NFS),and the largest difference of SOC between elevations within the transect occurred on the WFS,where the topsoil organic carbon at2884m was about1.24times larger than that at2949m.The SOC density at0–60cm showed a similar distribution pattern compared with the SOC concentration along the aspect gradients (Table2).The amount of organic carbon stored in the soil on the NFS (33.64±5.82kg m−2)was about3.2,2.9and1.9times larger than on the SFS(10.52±2.13kg m−2),SWFS(11.74±1.65kg m−2)and WFS(17.72±3.43kg m−2).In addition,the organic carbon stored at 0–20cm was much higher than at20–40and40–60cm at all sampling positions.Table2Distribution patterns of SOC(mean±S.D.)Transect Elevation(m)Depth(cm)Bulk density(g cm−3)SOC concentration(g kg−1)SOC density(kg m−2)SOC density in0–60cmSFS2962±500–10 1.04±0.0924.10±3.69 2.49±0.379.93±1.5410–20 1.03±0.0420.28±4.31 2.07±0.3920–40 1.09±0.0514.96±2.48 3.26±0.5440–60 1.13±0.089.38±2.32 2.11±0.592943±390–10 1.12±0.0824.49±4.92 2.75±0.599.73±1.8410–20 1.14±0.1318.61±4.01 2.13±0.5520–40 1.13±0.1612.73±3.19 2.90±0.9840–60 1.18±0.188.59±2.45 1.96±0.402930±340–10 1.15±0.1126.45±8.00 2.97±0.6911.92±2.4010–20 1.09±0.1321.64±4.96 2.29±0.3320–40 1.09±0.1517.86±5.85 3.76±0.7840–60 1.10±0.1513.63±5.72 2.89±0.91SWFS2956±520–10 1.02±0.0431.12±3.78 3.17±0.3511.16±1.0510–20 1.01±0.0622.93±4.99 2.27±0.4320–40 1.05±0.1416.11±3.49 3.30±0.5140–60 1.10±0.2211.39±2.11 2.41±0.302930±450–10 1.09±0.0625.56±2.72 2.77±0.3011.06±0.9910–20 1.06±0.0620.22±1.65 2.14±0.2220–40 1.03±0.0816.80±2.30 3.48±0.6540–60 1.07±0.1112.53±1.46 2.66±0.272903±410–10 1.10±0.0430.31±5.57 3.33±0.5012.94±2.0210–20 1.06±0.0424.12±4.76 2.56±0.4720–40 1.04±0.0419.56±3.67 4.06±0.7740–60 1.04±0.0514.37±3.32 2.98±0.71WFS2949±470–100.91±0.1249.64±8.79 4.48±0.9416.88±2.6610–200.92±0.0933.61±7.62 3.06±0.4620–400.93±0.0528.78±7.51 5.32±1.1640–600.94±0.0621.48±6.27 4.03±1.102910±360–100.92±0.0951.09±12.51 4.61±0.9616.77±2.9410–200.97±0.0934.71±6.47 3.32±0.4720–400.95±0.0827.65±6.60 5.14±0.9340–600.95±0.0920.03±6.75 3.70±1.132884±470–100.87±0.1161.53±17.60 5.15±1.1819.52±4.1410–200.93±0.0843.34±11.55 3.92±0.8920–400.91±0.0631.98±8.41 5.73±1.5340–600.95±0.1525.20±8.49 4.71±1.71NFS2957±530–100.61±0.10106.07±12.34 6.36±1.1730.87±4.9210–200.65±0.1081.89±9.41 5.29±0.7020–400.80±0.2169.24±16.7910.65±2.1540–600.91±0.2149.17±19.648.57±3.352941±530–100.70±0.09112.94±20.947.78±1.5635.21±6.9810–200.86±0.1581.48±18.90 6.84±1.2220–400.88±0.1566.77±17.1211.45±2.6340–600.96±0.1347.17±11.089.15±2.872926±560–100.66±0.13106.32±25.727.06±1.9533.23±5.1610–200.78±0.1175.36±6.50 5.88±0.7420–400.82±0.1465.01±18.4110.21±2.4540–600.99±0.2153.08±18.8010.07±3.8997M.Zhu et al./Catena152(2017)94–1023.2.Effects of slope aspects and soil depths on SOCThe results of the GLM suggest that,at the hill scale,slope aspects,depths and elevations explained 80.19%,18.94%and 0.81%of the overall variation of SOC concentration (Table 3).In the MLM analysis,the over-all variation of SOC explained by slope aspects and depths was a bit higher than in the GLM analysis (Table 3).Although the effect of eleva-tions on SOC concentration was statistically signi ficant (P b 0.01),the variation explained by elevations was b 1%and 4%of that explained by aspects and depths,respectively.This demonstrated that,at the hillscale,the SOC distribution pattern can be well described with only as-pect and depth as the effect of elevations was tiny.3.3.SOC as functions of slope aspects and soil depthsAs the effect of elevation on SOC at the hill scale is trivial compared to the aspect and depth,we only used aspects and depths as indepen-dent variables in the construction of the pro file pedotransfer functions.Table 4Regression coef ficients of the SOC concentration vs.depth relations.HillTransectAspect (°)Cosine of aspectSOCconcentration =a −b ln(h )Coef ficient aCoef ficient b ASFS 180−1.00037.16 6.71SWFS 225−0.70736.63 6.13WFS 2700.00068.7710.66NFS 360 1.000152.6728.21BSFS 180−1.00039.55 6.53SWFS 225−0.70740.37 6.94WFS 2700.00094.4418.98NFS 360 1.000160.5527.25CSFS 180−1.00030.16 5.27SWFS 225−0.70744.747.65WFS 2700.00063.4911.45NFS3601.000130.9518.67Fig.2.Description of SOC concentration with azimuth angle of slope aspect and soil depth.(a1),(b1),and (c1)are regressions between SOC concentration and soil depth for Hills A,B and C,respectively;(a2),(b2),and (c2)are regressions between coef ficients of Eq.(2)and cosine of azimuth angle for Hills A,B,and C,respectively;(a3),(b3),and (c3)are the distribution of SOC concentration estimated by Eq.(4)for Hills A,B,and C,respectively.Table 3Effects of slope aspects,depths and elevations on SOC concentration at the hill scale.SourceDFGeneral linear model Mixed linear model F valueSS%F value SS%Aspect 11414.58⁎⁎80.191421.61⁎⁎80.39Depth 1334.12⁎⁎18.94335.06⁎⁎18.95Elevation 114.36⁎⁎0.8110.77⁎⁎0.61Residuals4280.060.05DF,F,and SS%are degrees of freedom,F-statistic,and proportion of the variance explained by variable;the results were obtained from general linear model (GLM)and mixed linear model (MLM)analyses.⁎⁎P b 0.01.98M.Zhu et al./Catena 152(2017)94–102During the construction phase,we found that for each sampling hill in this study,signi ficant logarithmic relations of the SOC concentration with depth were observed at each aspect (Fig.2a1–c1).The R 2values of the regression functions varied from 0.58to 0.76,0.53to 0.87,and 0.59to 0.74on Hills A,B and C,respectively (Fig.2a1–c1).As shownin Table 4and Fig.2,the regression coef ficients of the SOC concentration vs.depth relations increased with increasing cosine of azimuth angle of aspects,following the exponential function.The R 2values of the regres-sion functions (i.e.,Eq.(3))on each hill were 0.99for a ,and 0.98for b .The SOC density at a depth h increased with increasing cosine of the azimuth angle of the slope aspect,following an exponential function (Table 5,Fig.3a1–c1).The R 2values of the regression functions (i.e.,Eq.(6))varied from 0.47to 0.90on the three hills.We observed margin-ally signi ficant exponential relations of the coef ficients a and b in Eq.(6)with depth on Hills A and B,and signi ficant exponential relations on Hill C (Fig.3a2–c2).The R 2values of the regression functions (i.e.,Eq.(7))varied from 0.97to 0.99.The coef ficients of Eqs.(4)and (8)for the three hills are shown in Table 6.The linear fits for the measured and estimated SOC indicated that Eqs.(4)and (8)were able to successfully explain the variation of SOC by the depths and slope aspects,with the slopes of the regression lines being close to 1,and the R 2values larger than 0.80(Fig.4).In addi-tion,we predicted the SOC density at 0–5,0–15,0–30,and 0–50cm on the SFS and NFS based on Eq.(8),and compared the estimates with pre-vious observations from Chen et al.(2016).The results showed that Eq.(8)was able to successfully predict the SOC density at different soil layers on the SFS and NFS observed in their study (Fig.5).ThelinearFig.3.Description of SOC density with azimuth angle of slope aspect and soil depth.(a1),(b1),and (c1)are regressions between SOCD h and the cosine of the azimuth angle for Hills A,B and C,respectively;(a2),(b2),and (c2)are regressions between coef ficients of Eq.(6)and soil depth for Hills A,B,and C,respectively;(a3),(b3),and (c3)are SOC density at different soil layers estimated by Eq.(8)for Hills A,B and C,respectively.Table 5Regression coef ficients of the SOCD h vs.aspect relations.HillDepth (cm)SOCD h =a e b cos(A )Coef ficient a Coef ficient b A50.4320.508150.3430.513300.2920.544500.2320.592B50.4990.455150.3680.543300.2920.647500.2170.780C50.4120.435150.3390.552300.2670.715500.2080.85899M.Zhu et al./Catena 152(2017)94–102fits between the estimates and observations from Chen et al.(2016)in-dicated that the R 2values were 0.94,0.93,and 0.93for Hills A,B,and C,respectively.According to Eqs.(4)and (8),we also predicted the distribution pat-tern on the three hills.The results show that the SOC concentration in-creased from the SFS to the NFS at all depths,and the rate of increase was lower on the SFS and NFS than on WFS (Fig.2a3–c3).In addition,the SOC density at 0–10,0–20,0–40,and 0–60cm increased from the SFS to the NFS,and the rate of increase was lower on the SFS and NFS than on the WFS (Fig.3a3–c3).4.Discussion4.1.Distribution patterns of SOCBoth SOC concentration and density increased from the SFS to NFS.Similar results were also obtained by other recent studies,which showed that the SOC concentration on the shady aspect (NFS)was sig-ni ficantly higher than other aspects (Sharma et al.,2011;Lenka et al.,2013;Huang et al.,2015).This distribution pattern was probably due to the heterogeneity of the soil temperature at the hill scale.In alpine re-gions,topographic obstructions and aspects affect the radiation distri-bution (Zhang et al.,2015).The potential annual direct incident radiation on the SFS was about 8%,37%and 93%higher than on the SWFS,WFS,and NFS,respectively (McCune and Keon,2002).Higher ra-diation resulted in higher soil temperature,which led to faster decom-position of soil organic matter,and consequently less accumulation of the organic carbon in soil on the SFS compared with the NFS (Kirschbaum,1995).In addition,the cover of grasslands increased from the SFS to WFS,and the vegetation type transformed to forests on the NFS (Table 1).The differences in vegetation cover and typemay also contribute to the distribution patterns of SOC along the aspect gradients.On the SFS,SWFS and WFS in this study,the SOC concentration and density tended to be higher at the lower elevations along each transect.This result was different from other studies that showed that the SOC in-creases with increasing elevation (Leifeld et al.,2005;Chen et al.,2016).This may be associated with the soil erosion along each transect.In this study,sampling positions (i.e.,1and 2)at higher elevations were at the shoulderslope and backslope positions of each transect,and the lower elevations sampling positions were at the footslope positions.The upper slope positions were generally eroded while the lower positions were depositional (McCarty and Ritchie,2002;Papiernik et al.,2005).Soil nutrients tend to accumulate in these depositional areas (Heckrath et al.,2005;Papiernik et al.,2007),thus the SOC is generally higher at the lower slope positions (Zhang et al.,2013;Sun et al.,2015).In our study region,the SFS,SWFS and WFS were occupied by grass-lands with cover b 0.7and suffered from slight to moderate soil erosion (He et al.,1992),soil erosion along these transects may enhance the ac-cumulation of SOC at lower positions,and this enhancement may mask the effect of elevation on SOC.4.2.Effects of slope aspects and soil depths on SOCThe slope aspects and depths in our study strongly affected the dis-tribution pattern of SOC.The GLM suggested that,at the hill scale,the variation of SOC concentration explained by elevation was much less than that explained by the slope aspects.Similar results were also ob-tained in Qilian Mountains by Chen et al.(2016),who indicated that,at a catchment scale,the aspect was able to explain 68.16%of the overall variation in SOC density at a soil depth of 0–50cm while the elevation explained only 29.33%.However,in our study,the effect of elevation was much tiny compared with Chen et al.(2016).The trivial effect of el-evation on SOC in our study was thus associated with the low relative elevations (b 100m)of the transects.In addition,compared with the other studies (Sharma et al.,2011;Huang et al.,2015;Sun et al.,2015;Lozano-García et al.,2016),the difference of SOC between the SFS and NFS in this study was much larger (close to fourfold).This was associat-ed with the strong slope aspect effects in the forest steppe zone of the Qilian Mountains,where the vegetation patterns are closely related to slope aspect,and represent a mosaic of grasslands and forests (Fig.1b).The tremendous difference of SOC along the aspect gradients also indicated that,to accurately estimate SOC storage in semiarid alpine re-gions,the slope aspect should be suf ficiently considered in SOC predic-tionmodels.parison between observations and estimates of SOC concentration (a)and density (b).Table 6Coef ficients of Eqs.(4)and (8)for the three hills.PropertiesHillCoef ficients c 1d 1c 2d 2e 1e 2SOC concentration (g kg −1)A 68.8710.77911.8190.861B 82.0780.68714.8700.640C 65.5130.69010.8100.556SOC density (kg m −2)A 0.2000.2820.4680.033−0.0410.027B 0.1860.393 1.543−1.129−0.048−0.008C0.1410.3161.217−0.856−0.031−0.017100M.Zhu et al./Catena 152(2017)94–102。
温度监测中英文翻译
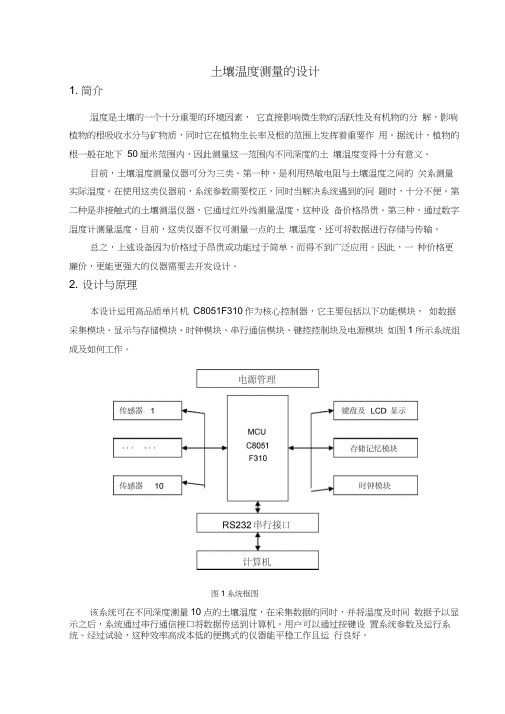
土壤温度测量的设计1. 简介温度是土壤的一个十分重要的环境因素,它直接影响微生物的活跃性及有机物的分解,影响植物的根吸收水分与矿物质,同时它在植物生长率及根的范围上发挥着重要作用。
据统计,植物的根一般在地下50厘米范围内,因此测量这一范围内不同深度的土壤温度变得十分有意义。
目前,土壤温度测量仪器可分为三类。
第一种,是利用热敏电阻与土壤温度之间的关系测量实际温度。
在使用这类仪器前,系统参数需要校正,同时当解决系统遇到的问题时,十分不便。
第二种是非接触式的土壤测温仪器,它通过红外线测量温度,这种设备价格昂贵。
第三种,通过数字温度计测量温度。
目前,这类仪器不仅可测量一点的土壤温度,还可将数据进行存储与传输。
总之,上述设备因为价格过于昂贵或功能过于简单,而得不到广泛应用。
因此,一种价格更廉价,更能更强大的仪器需要去开发设计。
2. 设计与原理本设计运用高品质单片机C8051F310作为核心控制器,它主要包括以下功能模块,如数据采集模块、显示与存储模块、时钟模块、串行通信模块、键控控制块及电源模块如图1所示系统组成及如何工作。
图1系统框图该系统可在不同深度测量10点的土壤温度,在采集数据的同时,并将温度及时间数据予以显示之后,系统通过串行通信接口将数据传送到计算机。
用户可以通过按键设置系统参数及运行系统。
经过试验,这种效率高成本低的便携式的仪器能平稳工作且运行良好。
2.1硬件设计在硬件设计中,系统可利用的部分包括C8O51F310单片机、DS18B2C数字温度传感器、ISL6292可编程锂电池充电管理芯片、NCP500电压管理芯片以及DS1302时钟芯片,它们通过相应的外围电路连接在一起,同时这几部分是系统的核心结构。
下面就介绍这些核心部分及其外围电路。
2.1.1高质量C8051F310单片机C8051F310是一款兼容8051指令集的完全集成的混合信号ISP型MCU芯片。
C8051F31C主要由CIP-51内核、外围模拟电路、数字I/O 口及电源模块组成。
211175514_CO2浓度增加和秸秆还田对黑土团聚体有机碳的影响
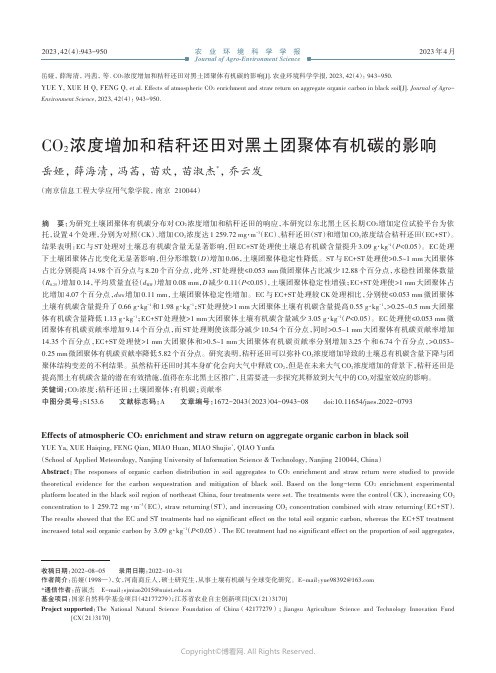
岳娅,薛海清,冯茜,等.CO 2浓度增加和秸秆还田对黑土团聚体有机碳的影响[J].农业环境科学学报,2023,42(4):943-950.YUE Y,XUE H Q,FENG Q ,et al.Effects of atmospheric CO 2enrichment and straw return on aggregate organic carbon in black soil[J].Journal of Agro-Environment Science ,2023,42(4):943-950.CO 2浓度增加和秸秆还田对黑土团聚体有机碳的影响岳娅,薛海清,冯茜,苗欢,苗淑杰*,乔云发(南京信息工程大学应用气象学院,南京210044)Effects of atmospheric CO 2enrichment and straw return on aggregate organic carbon in black soilYUE Ya,XUE Haiqing,FENG Qian,MIAO Huan,MIAO Shujie *,QIAO Yunfa(School of Applied Meteorology,Nanjing University of Information Science &Technology,Nanjing 210044,China )Abstract :The responses of organic carbon distribution in soil aggregates to CO 2enrichment and straw return were studied to providetheoretical evidence for the carbon sequestration and mitigation of black soil.Based on the long-term CO 2enrichment experimentalplatform located in the black soil region of northeast China,four treatments were set.The treatments were the control (CK ),increasing CO 2concentration to 1259.72mg·m -3(EC ),straw returning (ST ),and increasing CO 2concentration combined with straw returning (EC+ST ).The results showed that the EC and ST treatments had no significant effect on the total soil organic carbon,whereas the EC+ST treatmentincreased total soil organic carbon by 3.09g∙kg -1(P <0.05).The EC treatment had no significant effect on the proportion of soil aggregates,收稿日期:2022-08-05录用日期:2022-10-31作者简介:岳娅(1998—),女,河南商丘人,硕士研究生,从事土壤有机碳与全球变化研究。
【清华】Carbon and climate change_October 2016

Photosynthesis & heterotrophic decomposition: Effect of CO2 and temperature :
• Photosynthesis stimulated by
– CO2 –Temperature (at least in temporate and boreal areas)
Carbon, soils and climate change
15
Climate variability and extremes
• C sequestration in terrestrial ecosystems –actual weather and extremes more important than average climate
……. But many models predict future decline in C stock
Models disagree, particularly on sensitivity soil organic matter decomposition to climate change.
Carbon, soils and climate change
10
Modelled CO2 sinks (hindcasts)
Carbon, soils and climate change
Are sink strengths decreasing?
11
Increased atmospheric CO2 and temperature: Initial increase organic carbon stock ……..
Carbon, soils and climate change
红壤侵蚀地马尾松人工林恢复过程中土壤非保护性有机碳的变化_吕茂奎
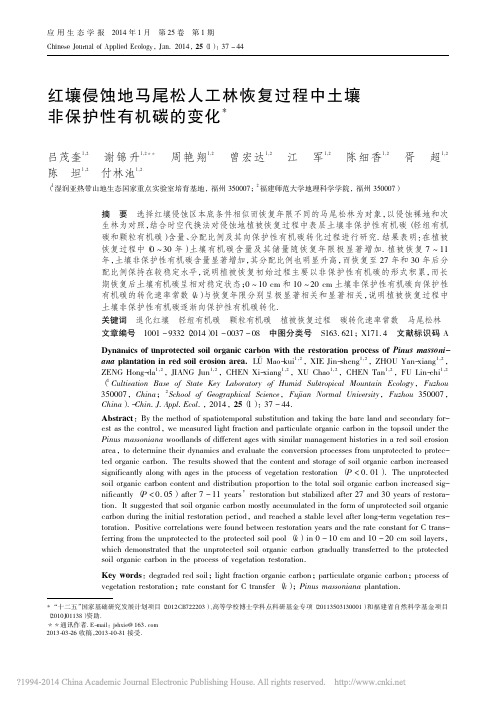
应用生态学报2014年1月第25卷第1期Chinese Journal of Applied Ecology,Jan.2014,25(1):37-44红壤侵蚀地马尾松人工林恢复过程中土壤非保护性有机碳的变化*吕茂奎1,2谢锦升1,2**周艳翔1,2曾宏达1,2江军1,2陈细香1,2胥超1,2陈坦1,2付林池1,2(1湿润亚热带山地生态国家重点实验室培育基地,福州350007;2福建师范大学地理科学学院,福州350007)摘要选择红壤侵蚀区本底条件相似而恢复年限不同的马尾松林为对象,以侵蚀裸地和次生林为对照,结合时空代换法对侵蚀地植被恢复过程中表层土壤非保护性有机碳(轻组有机碳和颗粒有机碳)含量、分配比例及其向保护性有机碳转化过程进行研究.结果表明:在植被恢复过程中(0 30年)土壤有机碳含量及其储量随恢复年限极显著增加.植被恢复7 11年,土壤非保护性有机碳含量显著增加,其分配比例也明显升高,而恢复至27年和30年后分配比例保持在较稳定水平,说明植被恢复初始过程主要以非保护性有机碳的形式积累,而长期恢复后土壤有机碳呈相对稳定状态;0 10cm和10 20cm土壤非保护性有机碳向保护性有机碳的转化速率常数(k)与恢复年限分别呈极显著相关和显著相关,说明植被恢复过程中土壤非保护性有机碳逐渐向保护性有机碳转化.关键词退化红壤轻组有机碳颗粒有机碳植被恢复过程碳转化速率常数马尾松林文章编号1001-9332(2014)01-0037-08中图分类号S163.621;X171.4文献标识码ADynamics of unprotected soil organic carbon with the restoration process of Pinus massoni-ana plantation in red soil erosion area.L Mao-kui1,2,XIE Jin-sheng1,2,ZHOU Yan-xiang1,2,ZENG Hong-da1,2,JIANG Jun1,2,CHEN Xi-xiang1,2,XU Chao1,2,CHEN Tan1,2,FU Lin-chi1,2(1Cultivation Base of State Key Laboratory of Humid Subtropical Mountain Ecology,Fuzhou350007,China;2School of Geographical Science,Fujian Normal University,Fuzhou350007,China).-Chin.J.Appl.Ecol.,2014,25(1):37-44.Abstract:By the method of spatiotemporal substitution and taking the bare land and secondary for-est as the control,we measured light fraction and particulate organic carbon in the topsoil under thePinus massoniana woodlands of different ages with similar management histories in a red soil erosionarea,to determine their dynamics and evaluate the conversion processes from unprotected to protec-ted organic carbon.The results showed that the content and storage of soil organic carbon increasedsignificantly along with ages in the process of vegetation restoration(P<0.01).The unprotectedsoil organic carbon content and distribution proportion to the total soil organic carbon increased sig-nificantly(P<0.05)after7-11years’restoration but stabilized after27and30years of restora-tion.It suggested that soil organic carbon mostly accumulated in the form of unprotected soil organiccarbon during the initial restoration period,and reached a stable level after long-term vegetation res-toration.Positive correlations were found between restoration years and the rate constant for C trans-ferring from the unprotected to the protected soil pool(k)in0-10cm and10-20cm soil layers,which demonstrated that the unprotected soil organic carbon gradually transferred to the protectedsoil organic carbon in the process of vegetation restoration.Key words:degraded red soil;light fraction organic carbon;particulate organic carbon;process ofvegetation restoration;rate constant for C transfer(k);Pinus massoniana plantation.*“十二五”国家基础研究发展计划项目(2012CB722203)、高等学校博士学科点科研基金专项(20113503130001)和福建省自然科学基金项目(2010J01138)资助.**通讯作者.E-mail:jshxie@163.com2013-03-26收稿,2013-10-31接受.在全球气候变化背景下,如何恢复退化土壤的固碳能力是当今生态学和土壤学关注的焦点问题和优先研究领域之一.造林和改善农林业的管理是增加退化土壤碳固定和减缓大气CO2浓度增加的重要方法[1],具有成本低、潜力大和可持续性等优点.目前我国土壤退化严重,增加退化土壤的碳汇已成为我国亟待解决的重大问题.我国南方红壤区是仅次于黄土高原的第二大侵蚀退化区,尤其是花岗岩发育的红壤大面积严重退化,碳密度低,固碳潜力大[2],增加侵蚀退化红壤的固碳能力对于我国气候变化国际谈判具有重要意义.福建省长汀县是我国南方典型的红壤侵蚀区.20世纪80年代至今,在当地政府的重视下,该区先后对该退化生态系统进行了大量的恢复与重建工作,昔日的“红色沙漠”如今已是满山翠绿,极大地改善了当地的生态环境[2].但是,有关植被恢复过程中土壤有机碳动态变化以及土壤有机碳固定的长期有效性等问题尚不清楚.因此,研究生态恢复过程中侵蚀地土壤有机碳库动态及调控过程对后续的生态恢复与保护具有重要价值.土壤有机碳(soil organic carbon,SOC)是表征土壤质量和健康状况的重要指标[3].但是在侵蚀地植被恢复过程中,土壤总有机碳积累的短期变化并不能指示生态恢复对土壤碳动态的影响.因此,识别更敏感的活性有机碳组分有助于阐明土壤有机碳的动态.土壤非保护性有机碳(unprotected SOC)是活性有机碳的一部分,由新近凋落的、部分分解的、与土壤矿质结合不紧的植物残体组成,主要包括轻组有机碳(light fraction organic carbon,LFOC)和颗粒有机碳(particulate organic carbon,POC)[4].非保护性有机碳对土地利用或植被覆盖变化的响应非常敏感,可以作为土壤有机碳库变化的灵敏指标[5-8].鉴于此,本研究应用土壤有机碳物理分组方法,结合时空代换法,探讨红壤侵蚀地不同恢复年限马尾松人工林表层土壤的轻组有机碳和颗粒有机碳含量及其分配比例的变化,以及土壤碳库的演变过程,以期为今后侵蚀地的生态恢复及重建提供科学依据.1研究地区与研究方法1.1试验地概况长汀县河田镇地处福建省西南部、汀江上游(25ʎ33'—25ʎ48'N,l16ʎ18'—116ʎ31'E),海拔300 500m.该地区属中亚热带季风气候,年均气温17.5 19.2ħ,年均降雨量1700mm,年均蒸发量1403mm,年均无霜期260d,年均日照时数1924.6h,≥10ħ积温4100 4650ħ.该地区地形为开阔的河谷盆地,四周被低山高丘所环抱;土壤主要为中粗粒花岗岩发育的红壤,可蚀性较高,原有地带性植被(常绿阔叶林)基本破坏殆尽,现有植被以马尾松(Pinus massoniana)次生林和人工林为主.花岗岩风化壳深厚,一般为10m,最深处达百米,降雨侵蚀严重,加之植被的大面积破坏,使得河田镇成为全国水土流失最严重的地区之一,许多地方的表层土壤已被剥蚀殆尽,后期植被恢复极为困难.本研究选取土壤母岩和成土条件相同、地形及地表生态过程相似的6个不同恢复年限的试验样地,其中7a与8a、10a与11a治理样地分别作为治理7a和10a的重复.以侵蚀裸地(CK1)和次生林(CK2)为恢复前、后的对照.样地治理前的土壤侵蚀状况以及土壤有机质含量与侵蚀裸地基本一致.因此,不同恢复年限马尾松林土壤有机质的差异主要由植被恢复和治理措施引起的植物生长条件及土壤生态过程的差异造成.试验地类型及基本概况为:1)侵蚀裸地(CK1):海拔315m,坡度为12ʎ,坡向NE35ʎ.土壤侵蚀度一般达强度以上,表层土壤流失殆尽,B层出露,表层土壤(0 20cm)有机质含量为1.4 2.5g·kg-1;地表植被以稀疏的马尾松小老头树(平均胸径4.3 6.6cm,平均树高3.1 4.6m)以及少量芒萁(Dicranopteris dichotoma)和野古草(Arundinclla setosa)为主;2)伯湖和乌石岽:海拔分别为318和378m,坡度18ʎ和22ʎ,坡向ES30ʎ和SW20ʎ,分别于2003和2004年开始治理,通过7 8年的封禁治理,林下植被覆盖度接近100%,马尾松平均胸径和树高分别为8.2cm和7.3m;3)石官凹和游坊:海拔分别为314和328m,坡度15ʎ和21ʎ,坡向SW20ʎ和ES25ʎ,分别于2000和2001年对原有低效马尾松林进行小水平沟整地,补植胡枝子(Lespedeza bicolor),后期封禁管护.经过10 11年的封禁治理,林下植被盖度分别达到85%和70%以上,马尾松平均胸径分别为10.4和7.5cm,树高分别为7.5和5.9m;4)水东坊:海拔310m,坡度18ʎ,坡向NE50ʎ,于1984年对其水平沟整地,补植黑荆,林下套种胡枝子,并进行封禁管护,林下植被覆盖度97%,马尾松平均胸径和树高分别为16.9cm和14.3m;5)八十里河:海拔310m,坡度18ʎ,坡向NE50ʎ,于1981年对其进行小水平沟整地,保留原有的马尾松等乔木,并在马尾松林下套种胡枝子、紫穗槐(Amorpha fruticosa).经过30年的人工恢复,林83应用生态学报25卷下植被覆盖度97%,马尾松平均胸径和树高分别为16.9cm和14.3m;6)次生林(CK2):海拔321m,坡度10ʎ,坡向WN10ʎ,主要树种为马尾松、木荷(Schi-ma superba)针阔混交林,林龄约70 110年(异林龄),林下植被覆盖度95%以上,马尾松平均胸径和树高分别为47.5cm和19.0m.1.2研究方法1.2.1土样采集2011年7月在每块试验地设3个20mˑ20m标准地,分别进行本底和生物量调查.在每个标准地上用内径5cm土钻按“S”型随机、等量、多点混合取样,取8 10个点,每个样地取3组0 10和10 20cm土层混合土样,带回室内,过2mm筛、风干后备用.土壤容重用环刀法测定.1.2.2土壤有机碳含量测定SOC含量采用浓硫酸-重铬酸钾高温外加热氧化法测定,土壤全氮采用全自动凯氏定氮法测定,轻组和颗粒有机碳氮采用碳氮元素分析仪(Elementar VarioEL III,德国)测定,SOC储量采用土壤容重和有机碳含量推算[9].1.2.3轻组有机碳的分离及分配比例参照Janzen 等[10]的分离方法,称取风干土样10g,放在100mL 离心管中,加入50mL NaI(1.7g·cm-3)重液,震荡30min后离心,然后用真空管吸取悬浮部分,通过微孔滤膜过滤,继续往离心管中加20 30mL NaI,重复上述过程2 3次,直至没有可见的轻组物质,至少用75mL0.01mol·L-1CaCl2冲洗以除去轻组中NaI,另加100 150mL去离子水冲洗轻组.然后将滤纸上的轻组洗到预先称量的器皿中,在60ħ下烘干,称量,计算烘干样品占总土壤样品质量的比例,再取出部分样品用于分析SOC含量.根据轻组质量和有机碳含量,计算LFOC数量.以LFOC数量除以SOC总量,得到LFOC的分配比例[11].1.2.4颗粒有机碳分离及分配比例称取风干土样20g,放入250mL塑料瓶中,加入100mL浓度为5g·L-1的六偏磷酸钠[(NaPO3)6]溶液,手摇15min,再用震荡器震荡18h(90r·min-1).将土壤悬液过53μm筛,用蒸馏水反复冲洗后,所有留在筛子上的物质在60ħ下烘干至恒量,计算该部分占整个土壤样品质量的比例.通过分析烘干样品中SOC,结合>53μm的颗粒质量计算POC数量.以POC数量除以SOC总量得到POC的分配比例[11].1.3数据处理土壤非保护性有机碳向保护性有机碳转化的速率常数(k)用下式计算:k=P/(TTp U)式中:U为非保护性有机碳碳库(颗粒与轻组有机碳的均值);P为保护性有机碳库(总有机碳与非保护性有机碳的差值);TTp为保护性有机碳周转时间,设为100年[11].所有数据处理和统计分析均在Excel2003和SPSS17.0软件下进行,方差分析采用LSD法,采用一元线性回归模型建立两个变量之间的相关关系,显著性水平设定为α=0.05.用Origin7.5和Excel2003软件作图.2结果与分析2.1植被恢复过程中土壤有机碳含量及其储量的变化由图1可以看出,马尾松人工林恢复过程中,侵蚀地表层(0 20cm)SOC含量和储量总体呈非线性增长,其中以0 10cm土层的增长更显著.在植被恢复7 11年时,与侵蚀裸地(CK1)相比,010cm SOC含量及其储量均极显著增加,而10 20cm SOC含量及其储量差异不显著.但在植被恢复7 11年间,0 10和10 20cm SOC含量及其储量随年限增加并无递增的趋势,且不同恢复年限之间无显著差异,可能与不同恢复年限侵蚀地的治理措图1植被恢复过程中土壤有机碳含量及储量Fig.1Storage and content of the soil organic carbon in theprocess of vegetation restoration(meanʃSD).CK1:侵蚀裸地Erosive bare land;CK2:次生林Secondary forest.下同The same below.不同小写字母表示同一土层不同恢复年限林地间的差异显著(P<0.05)Different letters in the same soil layer denoted sig-nificant difference between the woodlands with different restoration yearsat0.05level.931期吕茂奎等:红壤侵蚀地马尾松人工林恢复过程中土壤非保护性有机碳的变化施不同以及试验地间的差异有关;在植被恢复27年和30年后,0 10cm SOC含量及其储量均显著高于CK1及恢复7 11年的样地;而10 20cm土层SOC含量及其储量显著高于CK1,但与恢复7 11年的样地无显著差异.这说明植被恢复优先影响0 10cm土层,随着治理年限的增加,下层土壤SOC 进一步积累.在植被恢复30年后,SOC含量及其储量仍显著低于恢复后的次生林(CK2),说明侵蚀地仍有较大的固碳潜力.2.2植被恢复过程中土壤轻组有机碳及其分配的变化植被恢复过程中LFOC并未随恢复年限呈线性上升(图2).轻组含量变化幅度较大,尤其是恢复7 11年,0 10和10 20cm土层轻组含量从CK1(1.2 2.2g·kg-1)增加到3.4 10.6g·kg-1(表1),0 10cm土层LFOC含量也极显著增加,是CK1(0.69g·kg-1)的4.5 4.7倍;而10 20cm土层与CK1无显著差异.在植被恢复27和30年后,其LFOC含量与7 11年无显著差异,但在这一阶段LFOC含量随恢复年限呈上升趋势,且逐渐趋近于CK2.植被恢复过程中,LFOC占总有机碳的比例也呈现不同程度的变化.总体上,恢复7 11年土壤轻组有机碳的分配比例(LFOC/SOC)明显高于治理27年后(图2),0 10cm土层LFOC/SOC从CK1(29.1%)增加到36.6% 41.9%,且0 10和10 20cm土层的变化趋势一致.当植被恢复7 11年,LFOC很大程度上决定了土壤总有机碳含量,且以0 10cm最为明显,其决定了总有机碳的25.9% 49.0%.植被恢复27和30年后LFOC/SOC逐渐保持在一定范围内(17.2% 21.6%),说明随着恢复年限增加,土壤有机碳组分逐渐趋于稳定.2.3植被恢复过程中土壤颗粒有机碳及其分配的变化植被恢复过程中土壤POC与LFOC的变化趋势相同(图2),且POC含量略高于LFOC含量.在植被恢复7 10年时,0 10cm土层颗粒物含量大幅度增加,与CK1(3.7g·kg-1)相比,增加了4.4 8.3倍,与恢复27和30年样地间无显著差异(表1);然而,在整个植被恢复过程中,10 20cm土层颗粒物含量的变化相对较小,不同恢复年限的样地间均无显著差异.土壤POC含量也呈相同的变化趋势,恢复前11年土壤POC大量积累,从0.22 g·kg-1增至2.99g·kg-1;而恢复至27年后,POC 的积累速率相对减小.在植被恢复27和30年后,0 10和10 20cm土层POC含量均呈增加趋势,且0 10cm土层增加较显著,比CK1(0.75g·kg-1)增加了4.8倍以上.植被恢复过程中,土壤POC分配比例(POC/ SOC)呈现不同程度的变化.总体上,恢复7 11年图2植被恢复过程中土壤轻组和颗粒有机碳含量及其占总有机碳的比例Fig.2Contents of LFOC,POC and their proportions to total soil organic carbon in the process of vegetation restoration(meanʃSD).04应用生态学报25卷表1植被恢复过程中土壤轻组和颗粒有机质组分含量及其碳(C)含量Table1Carbon contents of soil light matter and particulate organic matter in the process of the vegetation restoration (g·kg-1)组分Fraction 土层Soillayer(cm)侵蚀裸地Erosive bare land(CK1)OM C恢复年限Restoration years7OM C10OM C27OM C30OM C次生林Secondary forest(CK2)OM CLFOM0 102.2318.39.9325.510.6301.97.4300.211.7276.614.2272.710 201.2292.73.4322.03.4304.01.8301.53.0265.77.1265.6 POM0 103.7200.619.4169.630.6135.227.2133.326.4185.533.0203.310 201.4160.59.1130.922.1125.216.595.315.2106.517.0133.0 LFOM:轻组有机质Soil light fraction matter;POM:颗粒有机质Particulate organic matter.OM:有机物质Organic matter.表2土壤总有机碳和非保护性有机碳与恢复年限及其相互之间的相关方程Table2Correlation equations between the total SOC,unprotect SOC and restoration years土壤有机碳SOC(g·kg-1)0 10cm10 20cm轻组有机碳LFOC(g·kg-1)0 10cm10 20cm颗粒有机碳POC(g·kg-1)0 10cm10 20cm年限Year(a)y=0.463x+3.340(r=0.972,P=0.016)y=0.144x+1.980(r=0.986,P=0.005)y=0.018x+2.277(r=0.670,P=0.225)y=0.011x+0.678(r=0.787,P=0.075)y=0.041x+2.377(r=0.929,P=0.027)y=0.017x+0.827(r=0.914,P=0.038)土壤有机碳SOC(g·kg-1)y=0.116x+1.499(r=0.791,P=0.071)y=0.126x+0.335(r=0.809,P=0.056)y=0.210x+0.946(r=0.993,P=0.000)y=0.223x+0.167(r=0.975,P=0.004)轻组有机碳LFOC(g·kg-1)y=0.725x+0.033(r=0.941,P=0.002)y=0.415x+0.104(r=0.861,P=0.022)图3土壤非保护性有机碳向保护性有机碳转化速率常数(k)与植被恢复年限的相关关系Fig.3Correlation between rate constant for C transfer from theunprotected to the protected soil pool(k)and restoration years.的变化幅度明显大于恢复27和30年后(图2),且两个土层变化趋势相似.植被恢复7 11年时,POC占据土壤有机碳的主要部分,0 10和10 20cm土层分别占总有机碳的38.3%和30.7%;随着植被恢复年限的增加,POC/SOC逐渐降低,恢复至27和30年后,两个土层POC/SOC分别减少至26.7%和29.5%,接近于CK2(25.3%和23.5%).这说明植被恢复27和30年后,土壤POC占SOC的比例逐渐趋于稳定.2.4恢复年限对土壤非保护性有机碳的影响相关分析表明,土壤轻组与颗粒有机碳含量在很大程度上取决于土壤总有机碳的含量(62.6%98.7%)(表2),且0 10cm土层POC含量与总有机碳含量呈极显著线性相关,说明LFOC和POC占据了总有机碳重要的部分;尤其是植被恢复7 11年,土壤总有机碳的13.2% 41.9%由非保护碳构成.两个土层有机碳含量与恢复年限呈显著或极显著线性相关.土壤非保护性有机碳含量与恢复年限之间呈线性相关(表2).其中POC含量与恢复年限之间达到显著相关水平.随恢复年限的增加,010cm土层非保护性有机碳向保护性有机碳的转化速率常数增加,而10 20cm土层达到显著相关水平(图3).这说明随着植被恢复年限增加,土壤中非保护性有机碳逐渐向保护性有机碳转化.3讨论3.1植被恢复对土壤有机碳及非保护性有机碳的影响本研究中,随着植被恢复年限的增加,SOC含量及其储量均呈增加趋势,且在植被恢复前11年,林地SOC含量及其储量发生不同程度的变化.这是因为植被恢复过程中,林地植被覆盖度明显增加,促进14 1期吕茂奎等:红壤侵蚀地马尾松人工林恢复过程中土壤非保护性有机碳的变化了马尾松生长.这一方面直接减少了SOC的流失,另一方面,通过凋落物及死亡根系的养分归还,增加了土壤有机物质的输入量[2,12].SOC积累的最大速率通常发生在土地利用方式或土地管理变化的初期[13],因此,在植被恢复初期SOC含量的变化较大.另外,生态恢复过程中土壤理化性质得到明显改善,微生物随恢复年限的增加发生显著变化,经过20 30年处于基本稳定[14].每年大量的枯枝落叶和营养元素等物质重新返回到生态系统中,且随着植被恢复演替的进行,有机物质输入量逐渐增多,为微生物提供了可利用的碳源、氮源,促进了微生物活性及微生物生物量的升高;另一方面,微生物数量的增加加速了枯落物及死细根的分解,促进养分归还,从而显著提高了SOC含量及其储量.LFOC对植被类型、土地利用方式、施肥及经营措施等非常敏感[15].本研究发现,植被恢复过程中土壤LFOC含量呈非线性增加趋势,可能与不同恢复年限样地治理措施及施肥制度的差异有关.其中0 10和10 20cm土层LFOC含量变化范围分别为0.69 3.24和0.35 1.13g·kg-1,均逐渐接近CK2,但相对低于川西典型植被类型(0.84 10.85 g·kg-1)[16]、暖温带次生林(0.86 15.21 g·kg-1)、落叶松人工林(0.54 14.17g·kg-1)[6]和亚热带格氏栲天然林(<9g·kg-1)[7].在植被恢复初期,施肥、整地等治理措施促进了马尾松和林下植被的快速生长[17],林地植被覆盖率明显提高,建立了凋落物和细根的物质循环途径,为轻组有机质提供了物质来源,因此土壤LFOC含量显著增加.此外,轻组有机碳占总有机碳29.1% 41.9%,接近落叶松人工林(29.3% 32.8%)[6],但高于暖温带次生林(19.0% 23.1%)[6]和川西典型植被类型(4.0% 13.4%)[16].植被恢复27年后LFOC分配比例开始下降,且保持在16.9%左右,接近于CK2(16.5%)和暖温带次生林(19.0% 23.1%)[6]的水平.这可能是植被恢复到一定程度后,土壤中物质来源与土壤微生物的分解保持在一个相对平稳的状态;植被恢复过程中土壤和轻组有机质C/N呈先增加后降低的趋势(图4),这反映了植被恢复过程中土壤有机碳的分解程度[9,18],同时,在分解过程中常发生N的微生物固定[19],因此土壤中轻组有机碳的积累速率明显高于总有机碳,引起植被恢复初期轻组有机碳分配比例较高及C/N比值升高.植被恢复过程中土壤POM与LFOM含量及其分配比例呈基本一致的趋势(图2).土壤POM含量(0.22 5.83g·kg-1)与山地常绿阔叶林和常绿落叶阔叶混交林(1.11 8.96g·kg-1)[16]、暖温带次生林(0.76 8.8g·kg-1)和落叶松人工林(0.89 10.64g·kg-1)[6]土壤颗粒有机碳含量相接近.颗粒有机碳占总有机碳的比例(17.0% 43.7%)接近于Degryze等[20](17% 38%)、Jr Garten[21](9% 27%)及Conant等[22](13% 31%)的研究结果.颗粒有机碳对气候条件、农业耕作与管理方式、土地利用类型变化及施肥等外界条件十分敏感[23],而且土壤有机质短期内的变化与波动主要发生在易氧化分解的那一部分[24-25],导致植被恢复初期土壤颗粒含量波动也较大.3.2土壤非保护性有机碳与植被恢复年限的耦合作用植被恢复过程中,土壤非保护性有机碳的积累速度明显高于土壤总有机碳,且土壤LFOM和POM 与土壤总有机碳呈线性正相关关系.土壤轻组和颗粒有机碳尽管是由不同测定方法获得,但两者之间呈显著线性相关关系(表2),表明它们在一定程度上能够指示土壤碳库及其稳定性的变化[6-8,26].因图4植被恢复过程中土壤、轻组和颗粒有机质C/N的变化Fig.4Changes of the C/N ratio of LFOM,POM and SOM in the process of vegetation restoration(meanʃSD).24应用生态学报25卷此,土壤非保护性有机碳可以反映植被恢复过程土壤有机碳的变化.植被恢复过程中土壤非保护性有机碳占总有机碳的比例先增加后趋于平缓,在恢复27年后土壤非保护性有机碳逐渐接近于CK2,说明土壤有机碳的输入与微生物分解过程逐渐达到相对平衡状态,植被恢复过程中土壤、轻组和颗粒有机质C/N的变化(图4)也可以证实这一点.因为高C/N的土壤有机质分解缓慢,微生物活性低,利于有机质的积累,而且土壤碳积累的最大速率通常发生在土地利用或土地管理变化的初期[13,18].另外,0 10和10 20cm 层土壤非保护性有机碳向保护性有机碳转化速率常数(k)与植被恢复年限显著相关(图3),即植被恢复过程中非保护性有机碳逐渐向保护性有机碳转化,说明在红壤侵蚀区,依靠人工生态修复和自肥作用来恢复土壤有机碳库质量,以及维持土壤有机碳的长期有效性是可能的.然而,土壤碳库需要漫长的时间才能达到平衡状态,因此仍需加强林地管理及合理疏伐来减少土壤水分胁迫,同时还应引入演替后续物种,促进植物群落的拓殖与更替[14].参考文献[1]IPCC.Land use,land-use change,and forestry//Wat-sonRT,Noble LR,Bolin B,eds.A SpecialReport ofthe Intergovernmental Panel on Climate Change.Cam-bridge:Cambridge University Press,2000[2]Xie JS,Guo JF,Yang ZJ,et al.Rapid accumulation of carbon on severely eroded red soils through afforestationin subtropical China.Forest Ecology and Management,2013,300:53-59[3]Ding G,Novak JM,Amarasiriwardena D,et al.Soil organic matter characteristics as affected by tillage man-agement.Soil Science Society of America Journal,2002,66:421-429[4]Six J,ConantRT,Paul EA,et al.Stabilization mecha-nisms of soil organic matter:Implications for C-satura-tion of soils.Plant and Soil,2002,241:155-176[5]Skjemstad JO,Taylor JA,Janik LJ,et al.Soil organic carbon dynamics under long-term sugarcane monocul-ture.Australian Journal of SoilResearch,1999,37:151-164[6]Wu J-G(吴建国),Zhang X-Q(张小全),Wang Y-H (王彦辉),et al.The effects of land use changes on thedistribution of soil organic carbon in physical fraction ofsoil.Scientia Silvae Sinicae(林业科学),2002,38(4):19-29(in Chinese)[7]Yang Y-S(杨玉盛),Liu Y-L(刘艳丽),Chen G-S(陈光水),et al.Content and distribution of unprotectedsoil organic carbon in natural and monoculture plantationforests of Castanopsis kawakamii in subtropical China.Acta Ecologica Sinica(生态学报),2004,24(1):1-8(in Chinese)[8]Jiang P-K(姜培坤).Soil active carbon pool under dif-ferent types of vegetation.Scientia Silvae Sinicae(林业科学),2005,41(1):10-13(in Chinese)[9]State Forestry Administration(国家林业局).Forest Soil Analysis Methods:Determination of Organic Matterin Forest Soil and Calculation of Carbon-NitrogenRatio(LY-T12371999).Beijing:China Standards Press,1999(in Chinese)[10]Janzen HH,Campbell CA,Brandt SA,et al.Light-fraction organic matter in soils from long-term crop rota-tions.Soil Science Society of America Journal,1992,56:1799-1806[11]Jr Garten CT,Post WM,Hanson PJ,et al.Forest soil carbon inventories and dynamics along an elevation gra-dient in the southern Appalachian Mountains.Biogeo-chemistry,1999,45:115-145[12]Li G-D(李国栋),Liu G-Q(刘国群),Zhuang S-Y (庄舜尧),et al.Changes of organic matter in soilsplanted Lei bamboo with different years.Chinese Journalof Soil Science(土壤通报),2010,41(4):245-249(in Chinese)[13]Smith P,Powlson DS.Glendining MJ,et al.Potential for carbon sequestration in European soils:Preliminaryestimates for five scenarios using results from long-termexperiments.Global Change Biology,1997,3:67-79[14]Xue S(薛?),Liu G-B(刘国彬),Dai Q-H(戴全厚),et al.Dynamic changes of soil microbial biomassin the restoration process of shrub plantations in loesshilly area.Chinese Journal of Applied Ecology(应用生态学报),2008,19(3):517-523(in Chinese)[15]Xie J-S(谢锦升),Yang Y-S(杨玉盛),Xie M-S(谢明曙),et al.Advance of research on light fraction or-ganic matter in soil.Journal of Fujian College of Forest-ry(福建林学院学报),2006,26(3):281-288(inChinese)[16]Xiang C-H(向成华),Luan J-W(栾军伟),Luo Z-S (骆宗诗),et al.Labile soil organic carbon distributionon influenced by vegetation types along an elevation gra-dient in west Sichuan,China.Acta Ecologica Sinica(生态学报),2010,30(4):1025-1034(in Chi-nese)[17]Xie J-S(谢锦升),Chen G-S(陈光水),He Z-M(何宗明),et al.Growth characteristics of Pinus massoni-ana under different improving patterns in degraded redsoil.Bulletin of Soil and Water Conservation(水土保持341期吕茂奎等:红壤侵蚀地马尾松人工林恢复过程中土壤非保护性有机碳的变化通报),2001,21(6):24-27(in Chinese)[18]Fissore C,Giardina CP,KolkaRK,et al.Temperature and vegetation effects on soil organic carbon qualityalong a forested mean annual temperature gradient inNorth America.Global Change Biology,2008,14:193-205[19]Recous S,Aita C,Mary B.In situ changes in gross N transformations in bare soil after addition of straw.SoilBiology and Biochemistry,1999,31:119-133[20]Degryze S,Six J,Paustian K,et al.Soil organic carbon pool changes following land-use conversions.GlobalChange Biology,2004,10:1120-1132[21]Jr Garten CT.Soil carbon storage beneath recently es-tablished tree plantation in Tennessee and South Caro-lina,USA.Biomass and Bioenergy,2002,23:93-102[22]ConantRT,Six J,Paustian K.Land use effects on soil carbon fractions in the southeastern United States.I.Management-intensive versus extensive grazing.Biologyand Fertility of Soils,2003,38:386-392[23]Xie J-S(谢锦升),Yang Y-S(杨玉盛),Chen G-S(陈光水),et al.Advances on soil particulate organicmatter.Journal of SubtropicalResources and Environ-ment(亚热带资源与环境学报),2009,4(4):43-52(in Chinese)[24]Biederbeck BO,ZentnerRP.Labile soil organic matter as influenced by cropping practices in an arid environ-ment.Soil Biology and Biochemistry,1994,26:1647-1656[25]Kalbitz K,Solinger S,Park JH,et al.Controls on the dynamics of dissolved organic matter in soils:A review.Soil Science,2000,165:277-304[26]Tang G-M(唐光木),Xu W-L(徐万里),Sheng J-D (盛建东),et al.The variation of soil organic carbonand soil particle-size in Xinjiang oasis farmland of differ-ent years.Acta Pedologica Sinica(土壤学报),2010,47(2):279-285(in Chinese)作者简介吕茂奎,男,1986年生,硕士研究生.主要从事森林水文与生态恢复研究.E-mail:228lmk@163.com责任编辑李凤琴44应用生态学报25卷。
Soil Biodiversity and Ecosystem Function
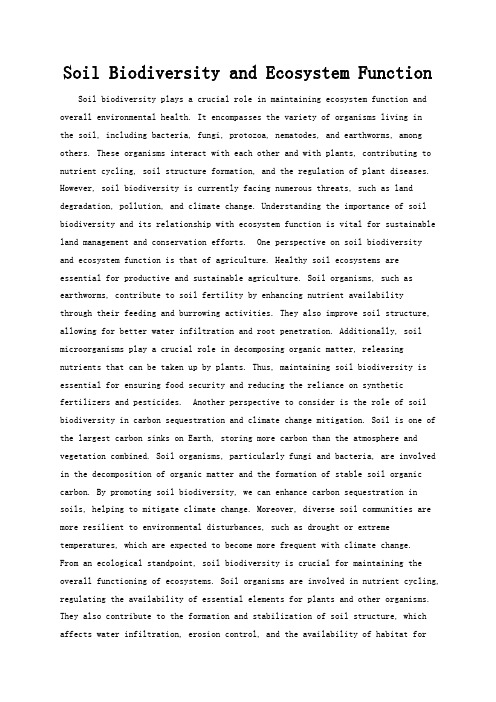
Soil Biodiversity and Ecosystem Function Soil biodiversity plays a crucial role in maintaining ecosystem function and overall environmental health. It encompasses the variety of organisms living inthe soil, including bacteria, fungi, protozoa, nematodes, and earthworms, among others. These organisms interact with each other and with plants, contributing to nutrient cycling, soil structure formation, and the regulation of plant diseases. However, soil biodiversity is currently facing numerous threats, such as land degradation, pollution, and climate change. Understanding the importance of soil biodiversity and its relationship with ecosystem function is vital for sustainable land management and conservation efforts. One perspective on soil biodiversityand ecosystem function is that of agriculture. Healthy soil ecosystems areessential for productive and sustainable agriculture. Soil organisms, such as earthworms, contribute to soil fertility by enhancing nutrient availabilitythrough their feeding and burrowing activities. They also improve soil structure, allowing for better water infiltration and root penetration. Additionally, soil microorganisms play a crucial role in decomposing organic matter, releasing nutrients that can be taken up by plants. Thus, maintaining soil biodiversity is essential for ensuring food security and reducing the reliance on synthetic fertilizers and pesticides. Another perspective to consider is the role of soil biodiversity in carbon sequestration and climate change mitigation. Soil is one of the largest carbon sinks on Earth, storing more carbon than the atmosphere and vegetation combined. Soil organisms, particularly fungi and bacteria, are involved in the decomposition of organic matter and the formation of stable soil organic carbon. By promoting soil biodiversity, we can enhance carbon sequestration in soils, helping to mitigate climate change. Moreover, diverse soil communities are more resilient to environmental disturbances, such as drought or extreme temperatures, which are expected to become more frequent with climate change. From an ecological standpoint, soil biodiversity is crucial for maintaining the overall functioning of ecosystems. Soil organisms are involved in nutrient cycling, regulating the availability of essential elements for plants and other organisms. They also contribute to the formation and stabilization of soil structure, which affects water infiltration, erosion control, and the availability of habitat forsoil-dwelling organisms. Furthermore, soil biodiversity plays a role in the suppression of plant diseases and the promotion of plant health throughmutualistic interactions. For example, mycorrhizal fungi form symbiotic associations with plant roots, enhancing nutrient uptake and providing plants with increased resistance to pathogens. However, soil biodiversity is under threat from various human activities. Intensive agricultural practices, such as the use of synthetic fertilizers and pesticides, can negatively impact soil organisms, reducing their diversity and abundance. Land degradation, including soil erosion and compaction, also leads to the loss of soil biodiversity. Pollution from industrial activities and improper waste disposal can contaminate soil, further compromising its biodiversity. Additionally, climate change poses a significant threat to soil biodiversity, as rising temperatures and altered precipitation patterns can disrupt the delicate balance of soil ecosystems. In conclusion, soil biodiversity is essential for maintaining ecosystem function and environmental sustainability. It plays a vital role in agriculture, carbon sequestration, and overall ecosystem health. However, soil biodiversity is currently facing numerous threats, including land degradation, pollution, and climate change. To safeguard soil biodiversity, it is crucial to adopt sustainable land management practices, reduce pollution, and mitigate climate change. By prioritizing the conservation and restoration of soil biodiversity, we can ensure the long-term health and productivity of our ecosystems.。
不同厚度砾石覆盖对土壤蒸发的影响

不同厚度砾石覆盖对土壤蒸发的影响周约1*,谢铁娜2(1.宁夏大学实验农场,宁夏银川750100;2.宁夏大学农学院,宁夏银川750021)摘要[目的]为了探求宁夏中部干旱地区最适宜的硒砂瓜覆砂厚度。
[方法]采用蒸发器整体称重法,以宁夏中卫南山台地区天然砂石为试材,研究了不同厚度砾石覆盖对土壤蒸发的影响。
[结果]砾石覆盖处理的土壤日蒸发量的大小顺序为CK>H1(5cm )>H2(8cm )>H3(10cm )>H4(15cm )。
同时,随着试验天数的增加,H3(10cm )和H4(15cm )处理的累积蒸发量的差别逐渐减小。
土壤总蒸发量随碎石覆盖厚度的增加呈现幂函数减少的变化趋势,拟合曲线的决定系数达到0.9255。
[结论]随着砂石覆盖厚度的增加,土壤日蒸发量逐渐减少,土壤含水量逐渐增加。
覆盖可以有效减小蒸发,且覆盖厚度越厚,对蒸发的抑制效果越明显。
在实施砾石覆盖处理时,应该适量增加覆盖厚度,阻止大量水分因蒸发而造成的水分损失。
当砾石覆盖厚度达10cm 以上时,砾石抑制蒸发的效果差别不大,因此10cm 的砾石覆盖厚度最适宜当地区域的自然条件。
幂函数方程Y =at b 可以用来模拟土壤总蒸发量随碎石覆盖厚度的变化。
关键词厚度;砾石覆盖;土壤蒸发基金项目宁夏大学自然科学研究基金项目(ZR1208);宁夏高等学校科学技术研究项目(NGY2014065)。
作者简介周约(1972-),男,宁夏中卫人,讲师,硕士,从事草地生态资源与环境方面的研究。
*通讯作者,E 鄄mail :****************。
收稿日期2015鄄06鄄20修回日期2015鄄08鄄06Effects of Different Thicknesses of Gravel Covering on Daily Soil EvaporationYue ZHOU 1鄢,Tiena XIE 21.Experimental Farm of Ningxia University,Yingchuan 750100,China;2.School of Agriculture,Ningxia University,Yingchuan 750021,ChinaSupported by Natural Science Research Foundation Item of Ningxia University,China (ZR1208);Science and Technology Research Item of Ningxia Colleges and Universities,China (NGY2014065).鄢Corresponding author.E 鄄mail:****************Received:June 20,2015Accepted:August 6,2015AAgricultural Science &Technology,2015,16(10):2347-2349,2353Copyright 訫2015,Information Institute of HAAS.All rights reservedResources and EnvironmentSoil evaporation is the process that water in soil enters into the air via rise and vaporizationfrom soil surface,and is a special stage of soil water movement process.In arid region ,rainfall is less,and evaporation causes loss of a large amount of water,thereby restricting improvement of dry agricultural crop field.Because gravel cover plays sig 鄄nificant roles in improving soil environ 鄄ment,increasing crop yield and raising crop quality,the technology is widely applied in agricultural production field of many countries and regions [1].A lot of researches show that as a kind of traditional cover technology,gravel cover can significantly decrease soil evaporation.The test aimed to study the impact of local natural gravel onsoil evaporation under different cover thicknesses,contrast change process of soil water evaporation under differ 鄄ent thicknesses of gravel cover,and analyze cumulative evaporation of soil under different thicknesses of gravel cover.Materials and MethodsTested materialsThe tested soil was taken from Yanghuang No.2Pump Station,Nan 鄄shantai,Letai Village,Yongkang Town,Shapotou District,Zhongwei City of Ningxia.Soil texture was sandy soil.After air drying,soil sample was crushed and screened by 2mm sieve.Particle size of gravel was about 2.5cm,and its cover thicknesses were 5,8,10and 15cm respectively,whichAbstract [Objective ]The research aimed to explore the most suitable gravel cover 鄄ing thickness for selenium sand melon in arid region of central Ningxia.[Method ]The natural gravel,which was from Nanshantai Region in Zhongwei City,Ningxia,was acted as test materials to study the effects of different thicknesses of gravel covering on daily evaporation using evaporator overall weighing method.[Result ]The daily evaporation capacity order of the gravel covering thickness was as follows:CK>H1(5cm)>H2(8cm)>H3(10cm)>H4(15cm).Meanwhile,with the increase of test days,the difference of cumulative evaporation capacity between H3(10cm)and H4(15cm)decreased gradually.Soil evaporation capacity reduced at the pow 鄄er function with the increase of gravel covering thickness,and the decision coeffi 鄄cient of the fitted curve reached to 0.9255.[Conclusion ]With the increase of gravel covering thickness,evaporation capacity of soil reduced gradually,and the soil water content increased gradually.Gravel covering could effectively reduce the evapora 鄄tion.The thicker of covering,the more obvious inhibition effect on evaporation.The thickness of covering should increase moderately to prevent moisture loss from e 鄄vaporation.Gravel inhibition effect on the evaporation wasn ’t obvious when the gravel covering thickness reached more than 10cm.10cm gravel covering was the most appropriate thickness for local natural condition.The soil evaporation capacity along with the change of gravel covering could be simulated with power function e 鄄quation Y =at b .Key words Thickness;Gravel covering;Soil evaporation2015Table 1Daily soil evaporation covered by different thicknesses of gravelTreatment Mean mm Maximum mm Minimum mm CV Skewness coefficient Kurtosis coefficient H10.32 2.360.080.68 4.0317.46H20.28 2.1200.64 3.9516.99H30.21 1.3900.74 3.3813.36H40.21 1.6300.63 3.8616.43CK0.906.760.250.684.2619.16were showed by H1-H4.Test methodsTo simulate evaporation,evapo 鄄rator used bucket made by PVC pipe bought from market with the diameter of 20cm and the height of 50cm.Par 鄄ticle size of gravel was about 2.5cm,and its cover thicknesses were 5cm (H1),8cm (H2),10cm (H3)and 15cm (H4)respectively.Meanwhile,a blank control (CK)was set,and each thickness had three repetitions.In the test,we should guarantee upper sur 鄄face alignment of bucket.At the bot 鄄tom of bucket,there was a layer of spun yarn,and it was clipped into ro 鄄tating screw thread of cover at the bot 鄄tom of bucket.Soil column was put in 鄄to plastic basin with water for 2days,and reached the saturation status via capillary action of soil.Then,it was set statically for 48hours.After excess water was discharged,different thick 鄄nesses of gravel were covered on soil surface.At 30cm of height over each soil column,a 200W of bulb was set to provide heat for evaporation.Indoor temperature and relative humidity were measured by dry and wet thermometer.Via daily weighing,soil evaporation was measured.The light was turned on for 8hours (09:00-17:00)every day.During whole test period,we should notice ventilation.Electronic balance with 30kg of mea 鄄suring range and 1g of sensitivity was used to weigh.After soil evaporation started,each variation of soil column mass was soil evaporation mass E soil (kg)on the day.E soil was converted into stan 鄄dard soil evaporation E (mm):E =E soil ×1000×10/(102π).Results and AnalysisSeen from Table 1,the mean ofdaily soil evaporation covered by gravel was significantly lower than that not covering.It illustrated that gravel cover could effectively improve physi 鄄cal property of soil underlying surface.Gravel can provide extra protection for soil surface,decrease runoff genera 鄄tion,protect soil moisture,prevent soil evaporation and guarantee moisture condition required by plant growth.In arid and semi 鄄arid regions,it plays the roles of improving regional eco 鄄envi 鄄ronment,regulating small climate and increasing utilization rates of water and soil resources to implement gravel cover.The sequence of daily soil evaporation covered by gravel was H1>H2>H3>H4,showing that as gravel cover thickness increased,daily soil evaporation gradually decreased,and soil moisture gradually increased.It was clear that when implementing gravel cover,we should appropriately increase cover thickness to hinder wa 鄄ter loss by evaporation,and promote sustainable development of dry agri 鄄culture.The coefficient of variation (CV )reflects dispersion degree of random variable (soil water evaporation).When CV >1,variable is strong varia 鄄tion.When 0.1<CV ,variable is moder 鄄ate variation.When CV <0.1,variable is weak variation.By analyzing CV of daily soil evaporation,it was obtained that CV of daily soil evaporation under different thicknesses of gravel coverand not covering conditions was all more than 0.1,showing as moderate variation.CV difference among treat 鄄ments was not large.Skewness coefficient illustrates symmetry degree of variable revolving mean,and Kurtosis coefficient illus 鄄trates steepness and flatness degrees of variable distribution.Both Skewness coefficient and Kurtosis coefficient of daily soil evaporation not covered by gravel were higher than gravel cover.It illustrated that symmetry and flatness of daily soil evaporation distribution not covered by gravel were worse than gravel cover,that is,extreme value of soil evaporation had larger impact on the treatment not covered by gravel.Seen from Table 2,cumulative soil evaporation among CK and differ 鄄ent treatments had significant differ 鄄ence (P <0.05).Meanwhile,as gravel cover thickness increased,soil mois 鄄ture evaporation also significantly de 鄄creased.Seen from Fig.1,temporal change rule of daily soil evaporation between different thicknesses of gravel cover and not covering was basically con 鄄sistent,which was mainly affected by meteorological factors (temperature,humidity and wind velocity)and pre 鄄sented continuous fluctuation change.Change magnitude of soil evaporation covered by gravel was obviously smaller than that not covered,which sufficiently embodied the effects ofFig.1Temporal change of daily soil evaporation under differentthicknesses of gravel cover Fig.2Temporal change of cumulative evaporation under differentthicknesses of gravel cover23482015(Continued on page 2353)Table 2Cumulative soil evaporation at different periods under different thicknesses of gravel covermmTreatment 5d 10d 15d 20d 25d 30d 35d 40d 45d H1 1.91b 2.87b 4.78b 10.51b 11.46b 12.74b 13.22b 13.85b 14.49b H2 1.59b 2.55b 4.30b 9.39b 10.19b 11.46b 11.62b 12.10b 12.42b H3 1.75b 2.71b 3.98bc 7.32c 7.80c 8.76c 9.08bc 9.24c 9.55c H4 1.27b 2.23c 3.50c 7.32c 7.80c 8.60c 8.76c 9.08c 9.39c CK3.18a5.25a10.03a27.07a29.94a34.08a35.99a38.22a40.61aDifferent letters showed significant difference at the level of 0.05.Table 3Fitted results for temporal change of cumulative evaporation under differentthicknesses of gravel cover Treatment Fitting typeFitting equationR 2H1Linear regression Y =0.2792t +1.38000.9544Power regression Y =0.6382t ^0.81500.9568Polynomial regressionY =-0.0024t 2+0.3926t +0.77150.9603H2Linear regression Y =0.2460t +1.23100.9496Power regression Y =0.5078t ^0.84620.9478Polynomial regressionY =-0.0030t 2+0.3861t +0.47970.9611H3Linear regression Y =0.1743t +1.55170.9413Power regression Y =0.7295t ^0.67370.9544Polynomial regressionY =-0.0022t 2+0.2767t +1.00190.9535H4Linear regression Y =0.1841t +1.06490.9350Power regression Y =0.2882t ^0.93850.8912Polynomial regressionY =-0.0031t 2+0.3285t+0.29020.9566CKLinear regression Y =0.8099t +1.26840.9640Power regression Y =0.8218t ^1.01520.9575Polynomial regressionY =-0.0018t 2+0.8918t +0.82880.9644gravel cover layer maintaining soil moisture and preventing soil evapora 鄄tion.Seen from Fig.2,cumulative evap 鄄oration under different cover thick 鄄nesses was obviously smaller than that of bare soil,that is,gravel cover could effectively inhibit soil evapora 鄄tion and improve utilization efficiency of water.Meanwhile,cumulative evap 鄄oration decreased as gravel cover thickness increased.When gravel thickness was 5cm,cumulative evap 鄄oration was the maximum.When grav 鄄el thickness was 15cm,cumulative evaporation was the minimum.It was similar with test result of Kemper et al [2-3].Test results showed that grav 鄄el cover could effectively decrease evaporation.Moreover,the thicker the cover thickness,the more obvious the inhibition effect on evaporation.Mean 鄄while,as test days increased,the dif 鄄ference of cumulative evaporation be 鄄tween H3and H4gradually de 鄄creased.It illustrated that when gravel cover thickness reached over 10cm,inhibition effect of gravel on evapora 鄄tion had small difference.Therefore,10cm of gravel cover thickness was the most suitable for local natural condition.To clear temporal change char 鄄acteristics of cumulative evaporation,and provide convenient way for pre 鄄dicting cumulative evaporation under gravel cover,temporal change result of cumulative evaporation under differ 鄄ent thicknesses of gravel cover was fitted.Seen from Table 3,correlation coefficient of linear regression was all more than 0.93,and correlation coeffi 鄄cient of power regression was all more than 0.89,while correlation coefficient of polynomial regression was all more than 0.95.It illustrated that polynomial equation Y =at 2+bt could be used to simulate change of cumulative evapo 鄄ration as time,that is,cumulative evap 鄄oration increased at the two power function as time.Here,dependent variable Y was cumulative evapora 鄄tion,and independent variable t was evaporation time,while both a and b were equation coefficients.Seen from Fig.3,total soil evaporation presentedpower function of decrease as gravel cover thickness increased,and deter 鄄mination coefficient of the fitted curve reached 0.9255.It illustrated that pow 鄄er function equation Y =at b could be used to simulate change of total soil evaporation as gravel cover thickness.ConclusionUsing weighing method,temporal change process of soil evaporation under different thicknesses of gravel cover was studied.Research showed that as cover thickness increased,dai 鄄ly soil evaporation gradually de 鄄creased,and soil moisture gradually increased.It illustrated that when im 鄄plementing gravel cover,we should appropriately increase cover thickness to hinder mass loss of water and pro 鄄mote sustainable development of dry farming.Both Skewness coefficient and Kurtosis coefficient of daily soil evaporation not covered by gravel were worse than gravel cover.It illus 鄄trated that the impact of extreme value of soil evaporation on not covering was larger.Cumulative evaporation under different cover thicknesses was all smaller than that of bare land.Mean 鄄while,cumulative evaporation de 鄄creased as gravel cover thickness in 鄄creased.Cumulative evaporation was the maximum when cover thicknessFig.3The impacts of different gravel cover 鄄ing thicknesses on cumulative evap 鄄oration23492015(Continued from page 2349)Responsible editor:Ping SONGResponsible proofreader:Xiaoyan WUwas 5cm and was the minimum when cover thickness was 15cm.However,the difference of cumulative evapora 鄄tion between H3and H4gradually de 鄄creased.It illustrated that when gravelcover thickness reached over 10cm,inhibition effect of gravel on evapora 鄄tion had small difference.Cumulative evaporation under different thickness 鄄es of gravel cover presented two pow 鄄er function of increase as evaporation time,and correlation coefficient was allmore than 0.95.Total soil evaporation presented power function of decrease as gravel cover thickness increased,and determination coefficient of the fit 鄄ting curve reached 0.9255.References [1]WANG TS (王天送),SU HC (苏贺昌),YANG SW(杨世维).Water characteris 鄄tics of sandy soil in Lanzhou region (兰州地区砂田土壤的水分特征)[J].Agri 鄄cultural Research in the Arid Areas (干旱地区农业研究),1991(1):66-69.[2]KEMPER WD,NICKS AD,COREY AT.Accumulation of water in soil sunder gravel and sand mulches [J].Soil Sci 鄄ence Society of America Journal,1994,58(1):56-63.[3]WEN MX (温明霞).Experimental study on water motion of different soil and stone mixed media (不同土石混合介质水分运动的试验研究)[D].Yangling:Northwest Agriculture and Forestry Uni 鄄versity(杨凌:西北农林科技大学),2011.Responsible editor:Tingting XU Responsible proofreader:Xiaoyan WU angles of root 鄄soil composites of Atriplex canescens 鄄planted slope and Caragana korshinskii 鄄planted slope were almost the same as that of pure slope;compared with that of pure slope,the cohesions of the two kinds of root 鄄soil composites were increased significantly,and the cohesion of root 鄄soil composite of Atriplex canescens 鄄planted slope was higher than that of Caragana korshinskii 鄄planted slope.The calculation and analysis showed that the stability coefficient in 鄄crements of the two kinds of root 鄄soil composites were all decreased with the increase in slope gradient,and the stability coefficient increment of Atriplex canescens 鄄planted slope was larger than that of Caragana korshin 鄄skii 鄄planted slope.When the slope gradient exceed 鄄ed 55°,the reinforcement effect of roots of the two kinds of shrub on shallow slope basically reached a limit,suggesting that plant roots play a role in improving slope stability when the slope gradient was less than 55°.References[1]GARY DH,ANDREW TL.Biotechnicalslope protection and erosion control [M].New York:Van Nostrand Reinhold Company,1982.[2]YANG YC (杨亚川),MO YJ (莫永京),WANG ZF (王芝芳),et al .Experiment study on anti 鄄water erosion and shear strength of soil 鄄root composite (土体-草本植物根系复合体抗水蚀强度与抗剪强度的试验研究)[J].Journal of China Agri 鄄cultural University (中国农业大学学报),1996,1(2):31-38.[3]ZHOU XJ(周锡九),ZHAO XF(赵晓锋).Strengthening role of plants on shallow slope (坡面植草防护的浅层加固作用)[J].Journal of North Jiaotong University (北方交通大学学报),1995,19(2):143-146.[4]LIN DG,HUANG BS,LIN SH.3鄄D nu 鄄merical investigations into the shear strength of the soil 鄄root system of Maki 鄄no bamboo and its effect on slope sta 鄄bility [J].Ecological Engineering,2010,36:992-1006.[5]LI GR (李国荣),HU XS (胡夏嵩),MAOXQ(毛小青),et al .Numerical simulation of shrub roots for slope protection ef 鄄fects on loess area of northeast Qing 鄄hai 鄄Tibetan Plateau(青藏高原东北部黄土区灌木植物根系护坡效应的数值模拟)[J].Chinese Journal of Rock Me 鄄chanics and Engineering(岩石力学与工程学报),2010,29(9):1877-1884.[6]XIAO BL (肖本林),LUO SL (罗寿龙),CHEN J (陈军),et al .Finite elementanalysis of eco 鄄protection slope through roots (根系生态护坡的有限元分析)[J].Rock and Soil Mechanics (岩土力学),2011,32(6):1881-1885.[7]RAJESH RB,SHRIVASTVA K.Biologi 鄄cal stabilization of mine dumps:shear strength and numerical simulation ap 鄄proach with special reference to Sisam tree[J].Environment Earth Sci,2007,12(4):1121-1142.[8]JI JN (及金楠).Analysis of root 鄄soil me 鄄chanical interaction to study tree an 鄄chorage and soil reinforcement by roots (基于根-土相互作用机理的根锚固作用研究)[D].Beijing:Beijing Forestry Uni 鄄versity(北京:北京林业大学),2007.[9]JIANG ZQ (姜志强),SUN SL (孙树林),CHENG LF (程龙飞).Soil-reinforcing effect of roots and slope stability under protection of plants (根系固土作用及植物护坡稳定性分析)[J].Site Investiga 鄄tion Science and Technology(勘察科学技术),2005,4:12-14.[10]LIU XP(刘秀萍).Finite element methodnumerical simulation of forest roots re 鄄inforcement(林木根系固土有限元数值模拟)[D].Beijing:Beijing Forestry Uni 鄄versity(北京:北京林业大学),2008.[11]THOMAS RE,POLLEN -BANKHEADN.Modeling root 鄄reinforcement with a fiber 鄄bundle model and Monte Carlo simulation [J].Ecological Engineering,2010,36(1):47-61.[12]Northwest Institute of Plateau Biologyof Chinese Academy of Sciences(中国科学院西北高原生物研究所).Qinghai Flora(Volume II)(青海植物志(第2卷)[M].Xining:Qinghai People ’s Pub 鄄lishing House(西宁:青海人民出版社),1997.[13]CHEN ZY(陈仲颐),ZHOU JX(周景星),WANG HJ(王洪瑾).Soil Mechanics(土力学)[M].Beijing:Tsinghua University Press(北京:清华大学出版社),1994.[14]LIU XL (刘秀萍),CHEN LH (陈丽华),SONG WF (宋维峰).Study on the shear strength of forest root 鄄loess composite (林木根系与黄土复合体的抗剪强度试验研究)[J].Journal of Bei 鄄jing Forestry University(北京林业大学学报),2006,28(5):67-72.[15]XIE WL(谢婉丽),WANG JD(王家鼎),WANG YL (王亚玲).Triaxial tests study on deformation and strength characteristics of reinforced loess (加筋黄土变形和强度特性的三轴试验研究)[J].Advance in Earth Sciences (地球科学进展),2004,19(plus):333-339.2353。
Chemistry of Soil Analysis

Chemistry of Soil AnalysisSoil is a crucial part of our ecosystem, providing the foundation for plant growth and nourishment. The health of our soils is directly linked to the health of our environment, and as such, it is essential to understand the chemical makeup and characteristics of soils in different areas. In this article, we will explore the chemistry of soil analysis, discussing the various techniques and tools used to evaluate soil quality.Soil CompositionThe composition of soil is complex, and it can vary significantly from one location to another. Soil is composed of both organic and inorganic materials, including minerals, water, air, and various microorganisms. The organic components of soil are derived from decomposed plant and animal matter, while the inorganic components include minerals and nutrients that are essential for plant growth.The chemical composition of soil is critical in determining its quality and suitability for crop growth. The main chemical components of soil include:- Nitrogen – essential for plant growth and protein synthesis.- Phosphorus – vital for cell division, energy production, and root development.- Potassium – important for water and nutrient absorption, disease prevention, and maintaining overall plant health.- Calcium – essential for root growth and development.- Magnesium – critical for photosynthesis and energy production.- Sulfur – important for protein synthesis and the production of chlorophyll.Soil Analysis TechniquesThere are various techniques and tools used to analyze the chemical composition of soil. These include physical testing, chemical testing, and biological testing methods.Physical TestingPhysical testing of soil involves assessing its texture, structure, and porosity. These factors affect the ability of soil to retain water, nutrients, and air, which in turn affects the plant's ability to grow. Physical testing methods include:- Soil volumetric weight determination – measures the weight of soil per volume.- Soil particle size analysis – determines the distribution of different particle sizes in soil.- Soil permeability testing – evaluates how easily water can flow through soil.Chemical TestingChemical testing of soil aims to determine its nutrient content, pH level, and the presence of contaminants. Soil testing methods include:- Soil pH testing – determines the acidity or alkalinity of soil, which can affect nutrient availability and plant growth.- Nutrient analysis – measures the levels of essential plant nutrients such as nitrogen, phosphorus, and potassium.- Soil contamination testing – identifies the presence of heavy metals and other contaminants that can affect soil quality and plant growth.Biological TestingBiological testing of soil involves evaluating the microbial activity of soil, which can affect nutrient cycling and plant growth. Soil testing methods include:- Microbial biomass analysis – determines the amount of microbial activity in soil.- Enzyme activity testing – measures the activity of enzymes in soil, which can indicate the level of microbial activity.Soil Analysis BenefitsSoil analysis is essential for evaluating the health of soil and determining its suitability for crop growth. Regular soil testing can provide crucial information about soil nutrient levels, pH, and the presence of contaminants. This information can help farmers and gardeners make informed decisions about fertilization and planting, leading to healthier plants and higher yields.Another significant benefit of soil analysis is the ability to monitor soil quality over time. As soil health is impacted by factors such as weather, farming practices, and environmental contaminants, regular testing can detect changes in soil quality. This information can help identify issues early and prevent further damage to soil health.ConclusionSoil analysis is a critical tool for evaluating the chemical composition and health of soil. With a range of testing methods available, soil analysis helps farmers, gardeners, and environmentalists make informed decisions about soil management and crop growth. Regular soil testing can ensure soil health, promote sustainable agriculture, and safeguard the environment.。
土壤碳循环英语作文
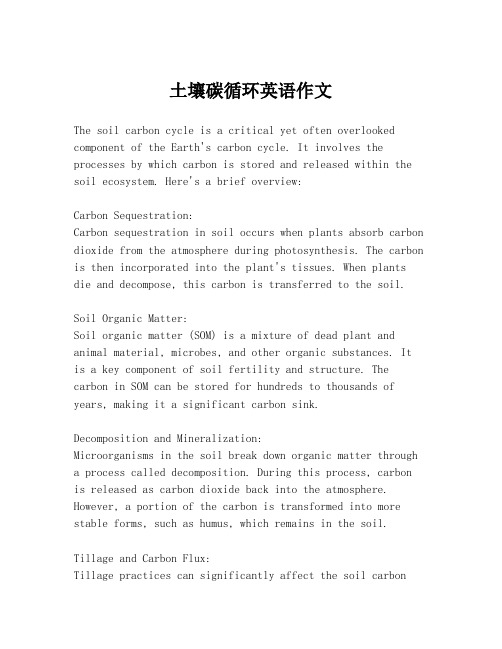
土壤碳循环英语作文The soil carbon cycle is a critical yet often overlooked component of the Earth's carbon cycle. It involves the processes by which carbon is stored and released within the soil ecosystem. Here's a brief overview:Carbon Sequestration:Carbon sequestration in soil occurs when plants absorb carbon dioxide from the atmosphere during photosynthesis. The carbon is then incorporated into the plant's tissues. When plants die and decompose, this carbon is transferred to the soil.Soil Organic Matter:Soil organic matter (SOM) is a mixture of dead plant and animal material, microbes, and other organic substances. Itis a key component of soil fertility and structure. The carbon in SOM can be stored for hundreds to thousands of years, making it a significant carbon sink.Decomposition and Mineralization:Microorganisms in the soil break down organic matter through a process called decomposition. During this process, carbonis released as carbon dioxide back into the atmosphere. However, a portion of the carbon is transformed into more stable forms, such as humus, which remains in the soil.Tillage and Carbon Flux:Tillage practices can significantly affect the soil carboncycle. Frequent tillage can accelerate the decomposition of SOM, leading to increased carbon dioxide emissions. Conversely, conservation tillage and no-till farming can help to preserve and even increase soil carbon storage.Climate Change Mitigation:Soil can act as a carbon sink, helping to mitigate the impacts of climate change. By promoting practices that increase soil carbon sequestration, such as agroforestry, cover cropping, and organic farming, we can enhance thesoil's capacity to store carbon.Conclusion:Understanding the soil carbon cycle is essential for sustainable agriculture and environmental conservation. It is a dynamic process that can be influenced by human activities. By adopting practices that promote soil health and carbon sequestration, we can contribute to the fight against climate change and ensure the long-term fertility of our soils.。
关于土的英语作文题目
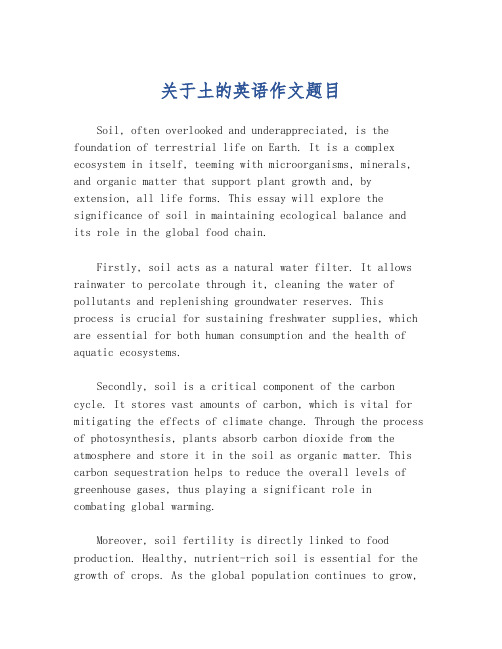
关于土的英语作文题目Soil, often overlooked and underappreciated, is the foundation of terrestrial life on Earth. It is a complex ecosystem in itself, teeming with microorganisms, minerals, and organic matter that support plant growth and, by extension, all life forms. This essay will explore the significance of soil in maintaining ecological balance andits role in the global food chain.Firstly, soil acts as a natural water filter. It allows rainwater to percolate through it, cleaning the water of pollutants and replenishing groundwater reserves. This process is crucial for sustaining freshwater supplies, which are essential for both human consumption and the health of aquatic ecosystems.Secondly, soil is a critical component of the carbon cycle. It stores vast amounts of carbon, which is vital for mitigating the effects of climate change. Through the process of photosynthesis, plants absorb carbon dioxide from the atmosphere and store it in the soil as organic matter. This carbon sequestration helps to reduce the overall levels of greenhouse gases, thus playing a significant role in combating global warming.Moreover, soil fertility is directly linked to food production. Healthy, nutrient-rich soil is essential for the growth of crops. As the global population continues to grow,maintaining and enhancing soil fertility becomes increasingly important to ensure food security. Sustainable agricultural practices, such as crop rotation and the use of organic fertilizers, can help preserve soil health and productivity.In addition to its agricultural significance, soil biodiversity is essential for the survival of countless species. It is home to a myriad of microorganisms, insects, and small animals that contribute to the decomposition of organic matter, nutrient cycling, and pest control. The loss of soil biodiversity can lead to a decline in ecosystem services, which are the benefits humans derive from nature, such as pollination, water purification, and climate regulation.Furthermore, soil is a non-renewable resource on a human timescale. It takes thousands of years to form a few centimeters of topsoil, and once it is degraded through erosion, compaction, or contamination, it is extremely difficult to replace. Therefore, it is imperative that we implement conservation measures to protect this precious resource.In conclusion, soil is a vital yet often undervalued resource that underpins life on Earth. It plays a critical role in water filtration, carbon sequestration, food production, biodiversity support, and the overall health of our planet. As we continue to face environmental challenges, it is essential that we recognize the importance of soil and take steps to preserve and enhance its quality for thebenefit of current and future generations.。
土壤碳常用英文单词
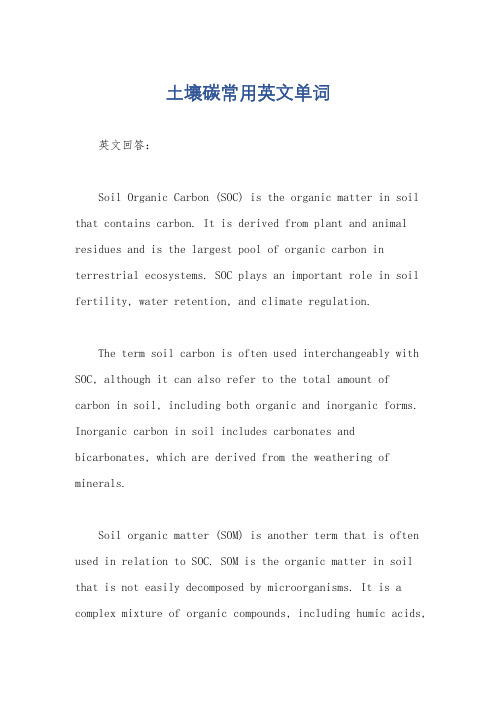
土壤碳常用英文单词英文回答:Soil Organic Carbon (SOC) is the organic matter in soil that contains carbon. It is derived from plant and animal residues and is the largest pool of organic carbon in terrestrial ecosystems. SOC plays an important role in soil fertility, water retention, and climate regulation.The term soil carbon is often used interchangeably with SOC, although it can also refer to the total amount of carbon in soil, including both organic and inorganic forms. Inorganic carbon in soil includes carbonates and bicarbonates, which are derived from the weathering of minerals.Soil organic matter (SOM) is another term that is often used in relation to SOC. SOM is the organic matter in soil that is not easily decomposed by microorganisms. It is a complex mixture of organic compounds, including humic acids,fulvic acids, and lignin. SOM plays an important role in soil structure and fertility.The following are some other common English terms used to refer to soil carbon:Soil organic carbon stock.Soil organic carbon pool.Soil organic carbon content.Soil organic carbon sequestration.中文回答:土壤有机碳 (SOC) 是土壤中含有碳的有机质。
读书报告4土壤碳固定对全球气候变化和粮食安全的影响
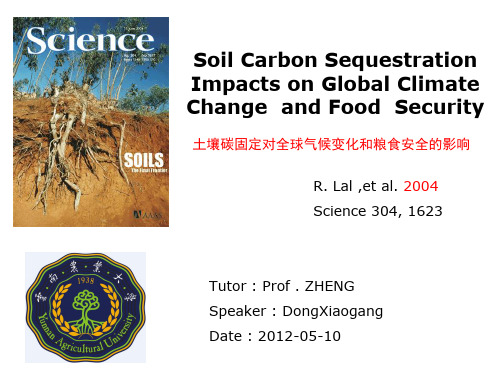
One ton of cereal residue contains 12 to 20 kg N, 1 to 4 kg P, 7 to 30 kg K, 4 to 8 kg Ca, and 2 to 4 kg Mg. Annually, 3 Gt of residues of grain crops are produced globally (table S3), which if recycled rather than removed for fuel and other uses, would improve soil quality and sequester C.
全球土壤碳固定总的潜力的估算值是非常广泛的,从低的0.4 to 0.6 Gt C/year (9) 到高的0.6 to 1.2 Gt C/year (13)。 因此,这种固定的潜力在容量和时间上是有限的。尽管如此,直到 我们能找到化石燃料的替代品时,土壤碳固定为我们争取了时间。
Some issues related to this strategy are as follows:
土壤碳固定意味着将大气中的CO2转移到长期存在的土壤库中并安全 地储存它,因此使碳不能立即释放到大气中。因此土壤碳固定意味着 通过精确的土地使用和推荐的管理措施(RMPs)增加了土壤有机和无 机碳库的容量。
ห้องสมุดไป่ตู้
2.Soil Carbon Sequestration
The SOC sequestration is caused by those management systems that add high amounts of biomass to the soil, cause minimal soil disturbance, conserve soil and water, improve soil structure, enhance activity and species diversity of soil fauna, and strengthen mechanisms of elemental cycling (Fig. 2, table S2).
Soil Carbon Sequestration

Soil Carbon SequestrationSoil carbon sequestration is an important process that involves the capture and storage of carbon dioxide from the atmosphere into the soil. This process is vital for mitigating the effects of climate change. The ability of soil to sequester carbon depends on various factors such as soil type, land use, and management practices. In this essay, I will discuss the importance of soil carbon sequestration, the factors that affect it, and the various management practices that can enhance it.Soil carbon sequestration is an essential process that helps to reduce the amount of carbon dioxide in the atmosphere. The process involves the conversion of atmospheric carbon dioxide into organic matter, which is then stored in the soil. The carbon is stored in the soil for long periods, which helps to reduce the amount of carbon dioxide in the atmosphere. This process is critical for mitigating the effects of climate change, which is one of the most significant challenges facing humanity today.The amount of carbon that can be sequestered in the soil depends on various factors such as soil type, land use, and management practices. Different soils have different capacities to store carbon. For instance, soils with high clay content can store more carbon than sandy soils. Similarly, land use practices such as deforestation and agriculture can have a significant impact on soil carbon sequestration. Deforestation leads to the loss of trees, which are essential for carbon sequestration, while agriculture can either enhance or reduce soil carbon sequestration depending on the management practices employed.Various management practices can enhance soil carbon sequestration. One of the most effective practices is the use of cover crops. Cover crops are crops that are planted to cover the soil during fallow periods. These crops help to prevent soil erosion, increase soil organic matter, and enhance soil fertility. They also help to sequester carbon by capturing carbon dioxide from the atmosphere and converting it into organic matter, which is then stored in the soil.Another effective practice is the use of conservation tillage. Conservation tillage involves reducing the amount of tillage or eliminating it altogether. This practice helps toreduce soil disturbance, which can lead to the loss of soil organic matter and soil erosion. It also helps to increase soil water retention, which is essential for plant growth and carbon sequestration.In conclusion, soil carbon sequestration is an essential process that helps to reduce the amount of carbon dioxide in the atmosphere. The amount of carbon that can be sequestered in the soil depends on various factors such as soil type, land use, and management practices. Different management practices can enhance soil carbon sequestration, including the use of cover crops, conservation tillage, and the use of organic fertilizers. These practices are critical for mitigating the effects of climate change, which is one of the most significant challenges facing humanity today. We must adopt these practices to ensure that we leave a better planet for future generations.。
Soil Biodiversity and Ecosystem

Soil Biodiversity and Ecosystem Soil biodiversity plays a crucial role in maintaining healthy ecosystems and supporting a wide range of essential functions. From providing nutrients for plant growth to regulating water and air quality, soil organisms are essential for the overall health of our planet. However, soil biodiversity is facing numerous threats, including land degradation, pollution, and climate change. These challenges highlight the importance of understanding and protecting soil biodiversity to ensure the sustainability of our ecosystems. One of the key benefits of soil biodiversity is its role in nutrient cycling. Soil organisms such as bacteria, fungi, and earthworms break down organic matter and release nutrients that are essential for plant growth. This process not only supports the productivity of agricultural systems but also helps maintain the balance of nutrients in natural ecosystems. Without healthy soil biodiversity, plants would struggle to obtain the nutrients they need, leading to reduced crop yields and ecosystem degradation. In addition to nutrient cycling, soil biodiversity also plays a crucial role in regulating water quality and quantity. Soil organisms help to improve soil structure, allowing for better water infiltration and storage. This, in turn, reduces the risk of erosion and helps to prevent flooding and droughts. By maintaining healthy soil biodiversity, we can ensure the availability of clean water for both humans and wildlife, as well as mitigate the impacts of extreme weather events caused by climate change. Furthermore, soil biodiversity is essential for carbon sequestration and climate regulation. Soil organisms store large amounts of carbon in the form of organic matter, helping to reduce the concentration of greenhouse gases in the atmosphere. By promoting the growth of plants and enhancing soil carbon storage, soil biodiversity plays a critical role in mitigating climate change. Protecting soil biodiversity is therefore essential for achieving global climate goals and ensuring the long-term sustainability of our planet. Despite the numerous benefits of soil biodiversity, it is facing increasing threats from human activities. Land degradation, caused by practices such as deforestation, overgrazing, and urbanization, is a major driver of soil biodiversity loss. Pollution from agricultural chemicals and industrial waste also poses a significant threat to soil organisms, leading to declines in biodiversityand ecosystem health. Additionally, climate change is altering soil conditions and disrupting the delicate balance of soil ecosystems, further endangering soil biodiversity. To address these challenges and protect soil biodiversity, it is essential to adopt sustainable land management practices and reduce our reliance on harmful chemicals. Conservation efforts, such as reforestation, soil restoration, and organic farming, can help to restore soil health and promote biodiversity. Education and awareness-raising initiatives are also crucial for fostering a deeper understanding of the importance of soil biodiversity and the need for its conservation. In conclusion, soil biodiversity is a vital component of healthy ecosystems and plays a critical role in supporting life on Earth. By recognizing the importance of soil organisms and taking action to protect and restore soil biodiversity, we can ensure the sustainability of our planet for future generations. It is essential that we work together to address the threats facing soil biodiversity and promote practices that support the health and resilience of soil ecosystems. Only through collective action and a commitment to conservation can we safeguard soil biodiversity and preserve the essential functions it provides for our planet.。
Soil Carbon Sequestration and Climate Change

Soil Carbon Sequestration and ClimateChangeSoil carbon sequestration is a critical process that has the potential to mitigate climate change by removing carbon dioxide from the atmosphere and storing it in the soil. This natural process involves the capture and storage of carbon in the soil through the decomposition of organic matter and the activities of soil organisms. As the global climate crisis continues to worsen, the role of soil carbon sequestration in combating climate change has gained increasing attention and significance. In this essay, we will explore the importance of soil carbon sequestration in the context of climate change, examining its potential benefits and challenges from various perspectives. From an environmental standpoint, soil carbon sequestration plays a crucial role in maintaining the balance of carbon in the atmosphere. With the increasing levels of carbon dioxide emissions from human activities such as industrial processes, deforestation, and the burning of fossil fuels, the concentration of greenhouse gases in the atmosphere has reached alarming levels. This has led to global warming and climate change, with devastating impacts on ecosystems, weather patterns, and sea levels. Soil carbon sequestration offers a natural solution to this problem by capturing and storing carbon in the soil, thus reducing the amount of carbon dioxide in the atmosphere and mitigating the effects of climate change. Furthermore, soil carbon sequestration has the potential to enhance soil fertility and agricultural productivity. By increasing the organic carbon content in the soil, this process can improve soil structure, water retention, and nutrient cycling, leading to better crop yields and overall soil health. In addition, it can help to reduce the need for synthetic fertilizers and pesticides, thereby promoting sustainable and environmentally friendly agricultural practices. This not only benefits farmers and food security but also contributes to the conservation of natural ecosystems and biodiversity. However, despite its potential benefits, soil carbon sequestration also faces several challenges and limitations. One of the key issues is the vulnerability of stored carbon to loss through land use changes, such as deforestation, urbanization, and agricultural expansion. When soils are disturbedor exposed to erosion, the stored carbon can be released back into the atmosphere, offsetting the gains achieved through sequestration. Moreover, the effectiveness of soil carbon sequestration can vary depending on soil type, climate, land management practices, and other factors, making it a complex and context-specific process. In conclusion, soil carbon sequestration holds great promise as anatural climate solution with multiple benefits for the environment, agriculture, and society. However, it is essential to address the challenges and limitations associated with this process in order to realize its full potential in mitigating climate change. By promoting sustainable land management practices, supporting agricultural innovation, and investing in research and education, we can harness the power of soil carbon sequestration to combat climate change and build a more sustainable future for generations to come.。
- 1、下载文档前请自行甄别文档内容的完整性,平台不提供额外的编辑、内容补充、找答案等附加服务。
- 2、"仅部分预览"的文档,不可在线预览部分如存在完整性等问题,可反馈申请退款(可完整预览的文档不适用该条件!)。
- 3、如文档侵犯您的权益,请联系客服反馈,我们会尽快为您处理(人工客服工作时间:9:00-18:30)。
Vol.12 No.4 2007 695-698Article ID: 1007-1202(2007)04-0695-04DOI 10.1007/s11859-006-0300-1Soil Organic Carbon Loss under Different Slope Gradients in Loess Hilly Region□JIA Songwei1,2, HE Xiubin1, WEI Fangqiang1†1.Key Laboratory of Mountain Hazards and Surface Process, Chinese Academy of Sciences/Institute of Mountain Hazards and Environment, Chengdu 610041, Sichuan, China;2.Graduate University of Chinese Academy of Sciences, Beijing 100049, ChinaAbstract:Based on field runoff plots observation and sample analysis, the effect of slope gradient on soil organic carbon loss was studied under natural rainfall conditions in loess hilly region. The results showed that with slope gradient increasing (from10° to 30°), the changing trend of soil erosion intensity (A) was A20°>A30°>A15°>A10°>A25°, suggesting that slope gradient between 20° and 25° was a critical degree ranger to exist. Soil organic car-bon loss was mainly influenced by soil erosion intensity, appearing the same trend as did soil erosion intensity with increasing slope gradient. Soil erosion results in organic carbon enrichment in sediment. Average enrichment ratios of five runoff plots varied from 2.27 to 3.74, and decreased with increasing erosion intensity and slope gradient. The decrease of surface runoff and soil erosion is the key to reduce soil organic carbon loss.Key words: soil erosion; soil organic carbon loss; slope gradient; carbon cycleCLC number:S 159.4Received date: 2006-09-02Foundation item: Supported by the National Natural Science Foundation of China (90502007) and the National Basic Research Program of China (973 Program 2007CB407206)Biography:JIA Songwei (1979-), male, Ph. D. candidate, research direction: soil erosion and soil organic carbon loss. E-mail: jiasongwei@† To whom correspondence should be addressed. E-mail: fqwei@ 0 IntroductionSoil organic carbon (SOC) pool is the largest and the most labile carbon pool on the earth’s surface sys-tem[1]. And total SOC amount is about 9.24×1016 g in the terrestrial ecosystem of China[2]. Soil erosion not only results in soil and water loss, but also takes away lots of SOC. In Iceland, Oskarsson et al. estimated that the amount of SOC loss was 1.2×1014-5.0×1014 g due to ac-cumulative historic soil erosion[3]. Harden et al. found that the loss of about 80% SOC was resulted from soil erosion on eroded cropland[4]. And in SOC loss process, part of organic carbon (OC) was mineralized and re-leased into the atmosphere[5]. Jacinthe et al. had also confirmed this point through artificial rainfall simula-tion[6]. So soil erosion and deposition may have a great effect on balancing the global atmospheric carbon budget through their impacts on the net exchange of carbon be-tween terrestrial ecosystems and atmosphere[7].In domestic previous studies, it mainly concerned in the loss of soil nitrogen, phosphorus and other nutrient elements. For example, Huang Manxiang et al.[8], Wang Hui et al.[9] and Zhang Yali et al.[10] studied the effects of different conditions on soil nitrogen loss through artifi-cial rainfall simulation experiment. Li Yuyuan et al.[11] and Yan Weijin et al.[12] studied the loss characteristic of soil phosphorus. But there are few reports about SOCloss at present. The Loess Plateau is an important carbon pool, as well as one of the most serious regions of soil erosion, especially in loess hilly region. Serious soil ero-sion can inevitably lead to large amounts of OC loss.Based on field runoff plots observation and sample analysis, this paper studied SOC loss characteristic under different slope gradients, and it may provide the basis to prevent SOC loss on sloping field.1 Materials and MethodsThe experiment was carried out at the Ansai Ex-perimental Station for Soil and Water Conservation, Chinese Academy of Science. The station is positioned at 109°19′ E and 36°51′ N, a typical loess hill and gully region with altitudes between 1 010 and 1 434 m. The main type of soil is loess loam soil. The climate is char-acterized by semiarid continental climate with annual average precipitation of 497 mm.The field runoff plots were established on sloping field at the Ansai Station in 1992 and in this experiment five plots were selected, composed of 10°, 15°, 20°, 25° and 30°. Each plot is 20 m in length and 5 m in breadth, surrounded with concrete block borders (extending 10 cm above the surface and 35 cm below the surface). A tank for runoff and sediment collection was installed at the outlet of each plot.During observation periods, prior to the rainy sea-son (in May), soil samples as basis were taken by core samplers. In each runoff plot, topsoil samples (0-15 cm depth) were collected by an “S” line and mixed for labo-ratory measurement.After each runoff event, the depth of runoff in each container was measured to calculate runoff volume, and 1 000 cm 3 of runoff water was sampled from each con-tainer after stirring and mixing. After being filtered, the remaining sediment was dried at low-temperature (45 ℃for 12 h drying) to measure sediment concentration. Then, the dried sediment samples were transported to the Insti-tute for laboratory analysis.OC content in soil and in sediment was determined by the oil bath-K 2Cr 2O 7 titration method [13]. Precipitationof each rainfall event was recorded with the help of rain gauge installed at the Ansai Experimental Station.2 Results and Analysis2.1 Soil Erosion under Different Slope GradientsSlope gradient is one of the important factors that affect slope erosion. In the same rainfall conditions, the overland flow can be very different and soil erosion in-tensity is also different due to different slope gradients. Total runoff amount (Q ) and erosion intensity (A ) of five storm events in 2002 were listed in Table 1, which shows that runoff mostly takes place in June and July, and it is in accordance with precipitation distribution in 2002 (Fig.1).Comparison was done between different plots for runoff amount and found that with increasing slope gra-dient, the changing trend of total runoff amount was Q 20°>Q 30°>Q 15°>Q 10°>Q 25°. So the turning point of runoff amount change is the plot with slope of 20°. The reason for this phenomenon was that with increasing slope gra-dient, the interaction area between rainfall and ground correspondingly reduced, and led to decrease precipita-tion in the unit area, and the infiltration of every plot wasdifferent as well. For each rain, with increasing rainfallFig.1 Distribution map of precipitationof study are a in 2002Table 1 Soil erosion characteristic under different plotsRunoff amount /m 3·km -2 Erosion intensity /t ·km -2Date Precipitation /mm I 30/mm ·min -110° 15° 20° 25° 30° 10° 15° 20° 25° 30° 2002.6.21 2002.6.28 2002.7.5 2002.7.30 2002.8.11 24.2 19.2 35.9 12.2 22.4 0.12 0.45 0.21 0.82 0.37 452.4 424.12 318.5 763.4 1 385.4 452.4480.75 089.41 159.22 318.5424.1593.86 474.82 770.92 827.4311.0424.11 979.2706.9848.2 1 413.7226.21 526.84 128.02 375.09.553.540.035.636.64.8 10.7 173.1 219.8 191.710.5 24.3 735.8 1 080.0 314.13.23.934.1181.034.511.916.773.01 565.0179.2Total 113.91.975 343.8 9 500.213 091.0 4 269.49 670.7175.1600.1 2 165.0 256.71846times, the changing trend of runoff amount gradually kept the consistent from 10°to 30°. And high rainfall intensity or precipitation could lead to runoff amount increase. For soil erosion intensity, its change trend was the same as runoff amount, it was A 20°>A 30°>A15°>A 10°>A 25°. Soil erosion intensity on slope of 20°was bigger than on slopes of 25°and 30°, except for storm events on June 21 and June 28. So the critical slope gra-dient was between 20°and 25°. Jin Changxing found that the critical slope gradient was between 24° a nd 29° by the equation of kinetic energy [14].2.2 Effects of Slope Gradient on SOC LossSOC loss causes a serious decline of land productiv-ity. SOC loss mainly depends on two approaches: one is the loss with sediment, and the other is that dissolve or-ganic carbon flows away with runoff. SOC loss amount in eroded sediment was listed in Table 2. It showed that slope gradient had an impact on SOC loss evidently and SOC loss increased by 1 time from 10° t o 15° a nd by 7.27 times from 10°to 20°. The changing trend of SOC loss amount was the same as that of erosion intensity. So SOC loss mostly was affected by erosion intensity.Table 2 SOC loss under different slope gradientsSOC loss/kg ·km -2Date Precipitation/mmI 30 /mm ·min -110° 15° 20° 25° 30° 2002.6.21 2002.6.28 2002.7.5 2002.7.30 2002.8.11 24.2 19.2 35.9 12.2 22.4 0.12 0.45 0.21 0.82 0.37 149 371 247 232 231 71 103 793 728 705 144 171 3 576 3 553 1 498 45 41 143 619 232 99 105 253 4 321 968 Total113.91.971 2302 4008 9421 0805 746Wang Baiqun et al . established the regressive model of soil nutrient loss and slope gradient based on the ob-servational data [15]. The model is as follows:()11exp ,S N a b s =i (1) Where S N is soil nutrient loss (kg ·km -2); 11,a b are coefficients; s is slope gradient (°).Based on observational data in 2002, they studied the relationship between SOC loss and slope gradient using this model, and validated it. Because the critical slope gradient is between 20° a nd 25° and SOC loss suddenly decreased on slope of 25°, so it was reasonablethat adopted some data with slope of ≤20°. The results are showed in Table 3.Table 3 Statistic results of SOC loss and slopegradients under different I 30 I 30/mm ·min -11a1br 0.2161.485 1.335 0 0.997 3 0.82 55.073 1.364 3 0.995 6 0.3796.090.935 90.993 7Table 3 shows that SOC loss was an exponent func-tion with slope gradient as slope gradient was less thanor equal to 20°. And the correlation coefficient reached to above 0.99 under different I 30.2.3 OC Enrichment in Eroded SedimentNutrient content in eroded sediment commonly is higher than in soil and it enriches in eroded sediment,because the particle size of sediment is less than soil par-ticle size due to the filtration of runoff during soil ero-sion process. Enrichment ratio (ER) is defined as the ratio of nutrient content in eroded sediment to nutrient content in soil. Tang Keli et al . compared the nutrient content in eroded sediment with that in sloping field and found that soil nutrient, such as organic matter, total ni-trogen etc, enriched in eroded sediment obviously [16]. Zhang Xingchang et al . reported that slope gradient had an impact on ER of organic matter in sediment, and ER decreased with slope gradient increasing [17].Table 4 shows that OC content in eroded sediment was higher than in soil evidently and ER ranged between 2.27 and 3.74. It also shows that ER had a decreasing trend with erosion intensity and slope gradient increasing. The main cause was that soil coarse particles easily lost with serious erosion intensity, but finer particles con-tained higher nutrient content, leading to the decrease of OC content in sediment and ER.Table 4 Effect of slope gradient on OC enrichment ratio OC content/g ·kg -1Plots/°Erosion inten-sity/t ·km -2 Soil SedimentER10 15 20 25 30175.1 600.1 2 165.0 256.7 1 846.02.232.322.552.582.328.34 7.22 6.72 7.8 5.26 3.743.112.643.022.27OC content in sediment was the average value3 ConclusionSOC is an important component of soil nutrient. And its loss not only leads to soil degradation, but also aggravates the atmospheric CO2 increase. This analysis shows that soil erosion is the main cause of SOC loss. And if strengthening soil and water conservation meas-ures were taken, SOC loss can be reduced. The results were as follows:① With slope gradient increasing, the changing trend of soil erosion intensity was A20°>A30°>A15°>A10° >A25°. The critical slope gradient of erosion intensity was between 20° and 25°.②The changing trend of SOC loss was the same to that of erosion intensity. So SOC loss mostly was affected by erosion intensity. SOC loss had an exponent function with slope gradient when slope gradient was less than or equal to 20°and the correlation coefficient was above 0.99.③Soil erosion results in OC enrichment in eroded sediment. The average ER varied from 2.27 to 3.74, and had a decreasing trend with increasing erosion intensity and slope gradient.[1]Pan Genxing, Li Lianqing, Zhang Xuhui. Perspectives onIssues of Soil Carbon Pools and Global Change—with Sug-gestions for Studying Organic Carbon Sequestration in Paddy Soils of China [J]. Journal of Nanjing Agricultural University, 2002, 25(3): 100-109 (Ch).[2]Wang Shaoqiang, Zhou Chenghu, Li Kerang, et al. Analysison Spatial Distribution Characteristics of Soil Organic Carbon Reservoir in China [J]. Acta Geographica Sinica, 2000, 55(5): 533-544 (Ch).[3]Oskarsson H, Arnalds O, Gudmundsson J, et al. OrganicCarbon in Icelandic Andosols: Geographical Variation and Impact of Erosion [J]. Catena, 2004, 56: 225-238.[4]Harden J W, Sharpe J M, Parton W J, et al. Dynamic Re-placement and Loss of Soil Carbon on Eroding Cropland [J].Global Biogeochemical Cycles, 1999, 13(4): 885-901.[5]Lal R. Soil Erosion and the Global Carbon Budget [J]. Envi-ronment International, 2003, 29: 437-450.[6]Jacinthe P A, Lal R, Kimble J M. Carbon Dioxide Evolutionin Runoff from Simulated Rainfall on Long-Term No-Till and Plowed Soils in Southwestern Ohio [J]. Soil & Tillage Re-search, 2002, 66: 23-33.[7]Liu S, Bliss N, Sundquist E, et al. Modeling Carbon Dynam-ics in Vegetation and Soil under the Impact of Soil Erosion and Deposition [J]. Global Biogeochemical Cycles, 2003, 17(2): 1074-1098.[8]Huang Manxiang, Zhang Shen, Zhang Guoliang, et al. Lossesof Nitrogen Nutrient in Overland Flow from Farmland in Bei-jing under Simulated Rainfall Conditions [J]. Acta Geo-graphic Sinica, 2003, 58(1): 147-154 (Ch).[9]Wang Hui, Wang Quanjiu, Shao Ming’an. LaboratoryExperiments of Soil Nutrient Transfer in the Loess Slope with Surface Runoff during Simulated Rainfall [J]. Transactions of the CSAE, 2006, 22(6): 39-44 (Ch).[10]Zhang Yali, Zhang Xingchang, Shao Ming’an, et al. Impact ofRainfall Intensity on Soil Mineral Nitrogen Loss by Runoff on Loess Slope [J]. Transactions of the CSAE, 2004, 20(3): 55-58 (Ch).[11]Li Yuyuan, Shao Ming’an. Effects of Phosphorus ApplicationMethods on Phosphorus Loss on Sloping Land under Simulated Rainfall [J]. Chinese Journal of Applied Ecology, 2002, 13(11): 1421-1424 (Ch).[12]Yan Weijin, Zhang Shen, Wu Shu’an, et al. BioavailablePhosphorus Loss and Its Prediction in Runoff under Simulated Rainfall Conditions [J]. Environmental Chemistry, 1999, 18(6): 497-506 (Ch).[13]Nelson D W, Sommers L E. A Rapid and Accurate Method forEstimating Organic Carbon in Soil [J]. Proceedings of the Indiana Academy of Science, 1975, 84: 456-462.[14]Jin Changxing. A Theoretical Study on Critical Erosion SlopeGradient [J]. Acta Geographica Sinica, 1995, 50(3): 234-239 (Ch).[15]Wang Baiqun, Liu Guobin. Effects of Relief on Soil NutrientLosses in Sloping Fields in Hilly Region of Loess Plateau [J].Journal of Soil Erosion and Soil and Water Conservation, 1999, 5(2): 18-22 (Ch).[16]Tang Keqiang, Zhong Zhongzi, Kong Xiaoling, et al. TheResearch of Soil Erosion and Soil Degradation on the Loess Plateau [J]. Environmental Science, 1984, 5(6) : 5-9 (Ch). [17]Zhang Xingchang, Shao Mingan. Enrichment of OrganicMatter and Nitrogen in Eroded Bedloads [J]. Chinese Journal of Applied Ecology, 2001, 12(4): 541-544 (Ch).[18]Yang Wenzhi, Yu Cunzu. Regional Control and Its Appraise-ment on the Loess Plateau [M]. Beijing: Science Press, 1992 (Ch).□References。