Giant planet formation from disk instability cooling and heating
A Survey for Massive Giant Planets in Debris Disks with Evacuated Inner Cavities
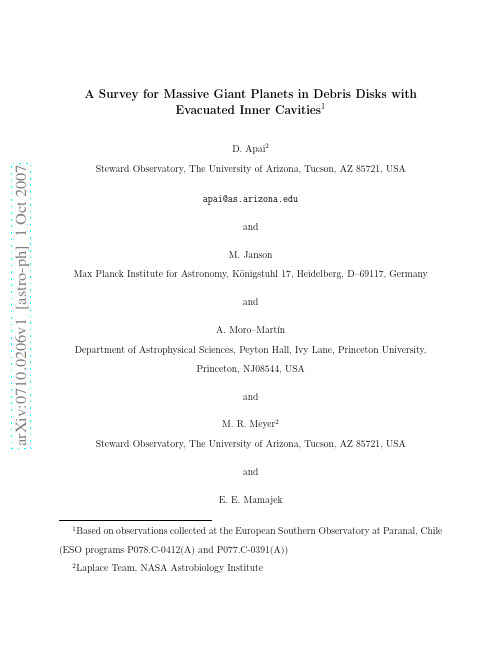
a rXiv:071.26v1[astro-ph]1Oct27A Survey for Massive Giant Planets in Debris Disks with Evacuated Inner Cavities 1D.Apai 2Steward Observatory,The University of Arizona,Tucson,AZ 85721,USA apai@ and M.Janson Max Planck Institute for Astronomy,K¨o nigstuhl 17,Heidelberg,D–69117,Germany and A.Moro–Mart´ın Department of Astrophysical Sciences,Peyton Hall,Ivy Lane,Princeton University,Princeton,NJ08544,USA and M.R.Meyer 2Steward Observatory,The University of Arizona,Tucson,AZ 85721,USAandE.E.MamajekHarvard–Smithsonian Center for Astrophysics,60Garden Street,MS-42,Cambridge,MA02138,USAandE.MasciadriINAF–Astrophysical Observatory Arcetri,ItalyandTh.HenningMax Planck Institute for Astronomy,K¨o nigstuhl17,Heidelberg,D–69117,GermanyandI.Pascucci,J.S.KimSteward Observatory,The University of Arizona,Tucson,AZ85721,USAandL.A.HillenbrandCalifornia Institute of Technology,Pasadena,CA91125,USAandM.KasperEuropean Southern Observatory,Karl–Schwarzschild–Str.2,D–85748Garching,GermanyB.BillerSteward Observatory,The University of Arizona,Tucson,AZ85721,USAReceivedABSTRACTThe commonality of collisionally replenished debris around main sequence stars suggests that minor bodies are frequent around Sun–like stars.Whether or not debris disks in general are accompanied by planets is yet unknown,but debris disks with large inner cavities–perhaps dynamically cleared–are considered to be prime candidates for hosting large–separation massive giant planets.We present here a high–contrast VLT/NACO angular differential imaging survey for eight such cold debris disks.We investigated the presence of massive giant planets in the range of orbital radii where the inner edge of the dust debris is expected.Our observations are sensitive to planets and brown dwarfs with masses>3to7Jupiter mass,depending on the age and distance of the target star.Our observations did not identify any planet candidates.We compare the derived planet mass upper limits to the minimum planet mass required to dynamically clear the inner disks.While we cannot exclude that single giant planets are responsible for clearing out the inner debris disks,our observations constrain the parameter space available for such planets.The non–detection of massive planets in these evacuated debris disks further reinforces the notion that the giant planet population is confined to the inner disk(<15AU).Subject headings:circumstellar matter–planetary systems–stars:individual(HD 105,HD377,HD107146,HD202917,HD209253,HD35850,HD70573,HD25457)1.IntroductionCollisionally replenished debris dust surrounds about10–20%of the main sequence Sun–like stars(e.g.Meyer et al.2007).Such widespread evidence for minor body collisions demonstrates that planetesimals orbit most stars.It is natural to ask whether or not rocky and giant planets are also present in these systems.No convincing correlation could yet be found between close–in exoplanets and the presence of debris(e.g.Moro-Mart´ın et al. 2007,but see Beichman et al.2005).However,the presence of massive giant planets has been often invoked to account for the observed azimuthal or radial asymmetries at large radii in many debris disks(e.g.Greaves et al.2005;Wilner et al.2002).While theoryoffers several alternative mechanisms(e.g.Takeuchi&Artymowicz2001;Wyatt2005), dynamical clearing of dust parent bodies by giant planets remains a feasible and exciting theoretical possibility(e.g.Moro-Mart´ın&Malhotra2005;Quillen2006;Levison et al. 2007;Morbidelli et al.2007).Examples for such possibly dynamically–cleared disks include two recently identified disks around the young Sun–like stars HD105(Meyer et al.2004)and HD107146 (Williams et al.2004).Both disks were found to exhibit strong excess emission at wavelengths longer than30µm,while displaying no measurable excesses shortward of20µm.The detailed analysis of the spectral energy distribution of HD105suggests that it is consistent with a narrow dust ring(<4AU)with an inner radius of∼42AU,if the dust grains emit like black bodies(Meyer et al.2004).Using a similar model Williams et al. (2004)showed that the excess emission from HD107146is consistent with arising from cold dust(T=51K)emitting as a single–temperature black body.The lack of measurable infrared excess shortward of25µm illustrates that the inner disk regions are well cleared of dust:for HD107146there is at most140×less warm dust(T=100K)than cold dust (T=51K,Williams et al.2004).Thefindings of the spectral energy distribution model forHD107146have been confirmed by direct imaging with the Hubble Space Telescope,that strengthen the case for a large featureless dust ring outside of an evacuated inner cavity (Ardila et al.2004).Recent high–contrast imaging surveys have hinted on the general scarcity of giant planets at such large separations(e.g.Masciadri et al.2005;Kasper et al.2007;Biller et al. 2007;Lafreniere et al.2007).Quantitative statistical analysis of the non–detections demonstrates that–at a90%confidence level–the giant planet population cannot extend beyond30AU if it follows a r0.2radial distribution,consistent with the radial velocity surveys.The statistical analysis suggests an outer cut–offfor the giant planet population at<15AU(Kasper et al.2007).If so,dynamically cleared cold debris disks may be the ssignposts for rare large–separation giant planets,ideally suited for direct imaging studies.In this paper we report on a VLT/NACO high–contrast imaging survey for large–separation giant planets around HD105,HD107146,and six other similar disks.Inthe following we will review the target stars and disks,the observations,followed by a comparison of our non–detections to lower planet mass limits set by dynamical clearing simulations.1.1.TargetsOur targets were selected from the sample of328Sun–like stars(0.7–2.2M⊙)targeted in the Formation and Evolution of Planetary Systems Spitzer Space Telescope Legacy program(FEPS,Meyer et al.2006).From this sample we identified8southern stars, which:a)display strong infrared excess emission at long wavelengths(λ>20µm);b)no measurable excess emission at shorter wavelengths;and,C)are young and close enough to permit the detection of planetary–mass objects within the inner radius of the cold debris.Table1gives an overview of the key parameters of the target stars.The typical lower mass limit for the debris in the systems is10−4to10−5M⊕,making these disks massive analogs of our Kuiper–belt(Meyer et al.2004;Kim et al.2005,Hillenbrand et al.,in prep).The disks of HD105and HD107146—included in our sample—have inner evacuated regions with an estimated radii of∼40AU and∼31AU(Meyer et al.2004;Williams et al.2004). The other six disks exhibit spectral energy distributions similar to HD105and HD107146. Based on the similarity of the excess emissions and the almost identical spectral types all eight disks are expected to have cleared–out inner disks of similar size.The only possible exception in this sample is HD202917,for which the re–calibration of the IRACfluxes after our VLT observations revealed a faint,but likely real infrared excess even at wavelengths shortward of10µm,suggesting that this inner disk may harbor small,but non–negligible amounts of warm dust.In the following we discuss briefly the results of the age determination for these sources as this has direct impact on the sensitivity of our observations to giant planets.A more detailed discussion of the ages of the whole FEPS sample will be presented in Hillenbrand et al.(in prep).We briefly summarize the upper and lower age estimates(t min and t max) for each star along with the most likely age t prob,where available.HD105has already reached the main sequence(t min=27Myr)and its chromospheric activity suggests a t max of 225Myr(Hillenbrand et al.,in prep.).Very likely a member of the Tuc–Hor moving group (Mamajek et al.2004)its t prob is30Myr(Hollenbach et al.2005).HD377is also a main sequence star(t min>25Myr)and the chromospheric activity suggests that t max=220Myr. The median of four other age indicators sets t prob=90Myr.For HD107146we adopt the age range of80–200Myr.HD202917is a likely member of the Tuc–Hor moving group (t min=t prob=30Myr)and its upper age limit is set by its Li–abundance,higher than that of the Pleiades(t max<100Myr).HD35850is suggested to be aβPic Moving Group member(t min=12Myr,Song et al.2003)and its observed rotation rate sets a reliableupper age limit of t max=100Myr(Hillenbrand et al.in prep.;cf.Barnes2007).HD70573 is among the few stars that are known to harbor both a debris disk and a giant planet. Setiawan et al.(2007)found an m2sini=6.1M J possible planet on a1.76–AU orbit.A combination of different age indicators suggest a t min=30Myr for HD70573and at prob=60Myr;Setiawan et al.(2007)quotes t max=125Myr.Based on Li–abundance and chromospheric activity,position on the color–magnitude diagram and the analysis of its space motions Mamajek et al.(in prep.)estimates that HD209253has t min=200Myr and t max<1.6Gyr with t prob=500Myr.HD25457is a member of the AB Dor moving group giving a very strong lower age limit(t min=50Myr,Zuckerman et al.2004).Luhman et al. (2005)derives an age of75–125Myr(we adopt t prob=75Myr),while the upper age limit is set by the chromospheric activity(t max=170Myr).2.Observations and Data ReductionOur8targets were observed with ESO’s Very Large Telescope using the NACO adaptive optics system(Lenzen et al.2003;Rousset et al.2003).The observations were carried out in service mode in late2006and early2007.The weather conditions were excellent with typical visual seeing of0.′′8and clear skies.We used the spectral differential imaging mode(SDI)of NACO in order to enhance the contrast for any methane–rich cold(T<1200K)companion(e.g.Lenzen et al.2004).The SDI mode uses two Wollaston prisms to split the incoming light rays into four beams of nearly identical light path.These rays pass through four narrow–bandfilters,two of which are identical.The three differentfilters(f1,f2and f3corresponding to1.575,1.600,and 1.625µm)probe the1.62µm methane feature and the adjacent continuum.Because the SDI mode uses the1024×1024–pixel S13camera of NACO with a13”×13”field of view, the simultaneous acquisition of4images in thisfield reduces the effectivefield of view toabout3”×3”.The achieved contrast,however,is not as good as predicted,probably due to the combined effect of read–out noise and a slightly lower Strehl ratio.With the reduced contrast our observations were sensitive only to planets beyond the1–3M J planet mass range.These planets–at the young ages of out targets–are too hot to display the1.62µm–methane feature(Burrows et al.2003).The lack of the methane feature results in almost identical planetfluxes in the f1,f2and f3filters,rendering the SDI technique inefficient.Instead,we opted to reduce the data taken in the f1filter(λc=1.575µm,δλ=0.025µm) in the angular differential imaging mode,i.e.without applying the spectral differential imaging step(e.g.,Mueller&Weigelt1987;Marois et al.2006;Kasper et al.2007).The data reduction was performed with a dedicated pipeline,as described in detail in Kellner (2005)and Janson et al.(2007).The frames taken at a given rotator angle were averaged and the collapsed frame corresponding to one angle was subtracted from the other.This procedure cancels out residual static or quasi–static features from the instrument,whereas any companion will remain as a combination of a positive and negative point source.The intensity of the residuals(at a certain separation from the primary)is characterized by taking the standard deviation in a9×9–pixel square(0.′′11×0.′′11≈(2.7λ/D)2)centered on that separation,at180evenly sampled angles,and taking the median of the results. This is repeated for all separations to create a radial profile of the error distribution in the image.When combined with the brightness of the primary,this yields the achieved contrast as a function of separation in thefinal image(see,Janson et al.2007for more details).Previous artificial planet tests on identical data sets processed with the reduction pipeline used here showed that3–sigma–bright sources would have been reliably identified as candidate planets(Janson et al.2007).Lacking any such detection,we used3–sigmafluxes as upper limits on the brightness of any companions to the target stars.In order to convert the achieved contrast in f1to the more commonly used H–band,we derived a conversion factor by comparing theflux densities in the twofilters in a simulated spectrum of a giant planet in the age and mass range probed by our observations(Burrows et al. 2003).3.ResultsThe NACO/ADI observations acquired high–contrast,high–resolution images of the 8target stars and their immediate environment(typically between10and70AU).In spite of the sensitive observations we could not identify giant planet candidates or any other point sources in the images.Our data analysis allows us to setfirm upper limits to the brightness of the sources that would have been identified as a candidate.We use the age estimates in Table1and planetary evolution models(Baraffe et al.2003)to convert the achieved sensitivities to planet masses.These limits are shown in Fig.1as a function of separation from the target stars for the lower and upper age limits of our stars.Using the high–contrast observations we can study the probable range of radii in these disks at which the dust debris and the parent body planetesimals reside.Our measurements exclude the presence of any brown dwarf companions for virtually all our targets at these radii.For HD209253,the oldest star in our sample,the images exclude companions down to the brown dwarf/giant planet mass boundary(13M J)at radii20AU or greater.For the the youngest source HD35850(12–100Myr)our observations exclude any giant planet companions down to3–4M J between25to45AU,if the star belongs to theβPic moving group as suggested by Song et al.(2003).For the other six sources our observations areTable1.Target parameters.Target R.A.(J2000)Dec.(J2000)V–mag.a Dist.[pc]a Sp.Type Ages:b t min/t prob/t maxa All magnitudes and distances from the Hipparcos catalog,except for the distance of HD70573,which is a main sequence–distance.b The age estimates are discussed in the text.Table2.Log of the observations.Target UT Dates NDIT×DIT a Frames per Angle Angles On–Source Strehl a DIT–Detector integration time;NDIT–number of integration averaged on–chip.typically sensitive to∼6M J at orbital radii>30–40AU.Note,that the dominant uncertainty of the upper limits stems from the difficulty of stellar age determination and from the poorly constrained initial conditions for giant planet evolution models.In particular,if shocks lead to efficient energy dissipation during the accretion phase,giant planets may start with much lower luminosities(e.g.Marley et al. 2007)than assumed by the hot–start models(e.g.Baraffe et al.2003;Burrows et al.2003).4.Discussion:Inside–Evacuated Debris Disks Without Massive GiantPlanets?If single planets are responsible for clearing out the inner disks in the observed systems, they will be located very close to the inner edge of the debris.Although the available data does not allow the direct measurement of the inner disk radii,simple black bodyfits to the spectral energy distributions provide reliable lower limits.We adopt these limits from Hillenbrand et al.(in prep.)and note that they range from6.2to28AU.Fig.1shows the limits for the individual sources(dashed lines).We stress again that these are lower limits —the real inner disk radii are probably somewhat larger.Given the range of detectable planet masses in Fig.1,we assess whether dynamical clearing by a less massive and therefore undetectable planet could still be a feasible mechanism to explain the lack of measurable quantities of warm dust,or on the contrary, if the dynamical clearing scenario may be rejected.Because our disks are devoid ofgas(Pascucci et al.2006)we use the dynamical models by Moro-Mart´ın&Malhotra (2005)to investigate the effect of a giant planet on the dust population.These models investigate the efficiency of dust particle ejection by gravitational scattering as a function of planet mass and planet location.In these models the dust particles are released fromFig.1.—The discovery space of the NACO observations(shaded),the minimum predicted planet masses required for a single planet to scatter out>90%of the planetesimals at a given radius(dotted lines)and the lower limits for the disk inner radius(dashed lines, Hillenbrand et al.,in prep.).The presence of planets within the shaded parameter range is excluded by our observations.The upper and lower shaded sensitivity curves mark the limits for a possible younger and older stellar age.For eachfigure the outer radius of the field of view is given in astronomical units in the lower right–hand corner.Note,that the reversing sensitivity curve for HD70573and HD209253is due to the reversal of the planetmass–luminosity curve in the corresponding evolutionary phase(Baraffe et al.2003).an outer belt of planetesimals and drift inward toward the central star under the effect of Poynting–Robertson drag,scattering as they cross the orbit of the planet and naturally creating a dust–depleted region inside its orbit.However,as shown in the upcoming study by Hillenbrand et al.(in prep.)all our disks are collision–dominated,as are bright debris disks in general(Wyatt2005).This means that the dust particles may not have time to drift too far from the parent bodies before getting eroded by collisions down to the blow-out size.Thus,in these collision–dominated disks the dust generally traces the location of the planetesimals.Therefore,we need to evaluate the effect of a giant planet on the planetesimal population rather than on the dust particles.Because gravitational scattering is a process independent of mass,the models of Moro-Mart´ın&Malhotra(2005)are also applicable to planetesimals as long as these can be considered to be“test particles”(i.e. their masses are negligible with respect to that of the planet).Using the above models we evaluate what is the mass of the least massive planet that can open a gap in the planetesimal distribution.In order to provide a good model for the evacuated inner gaps we require that the planet scatters out at least90%of the planetesimals.A dotted line in Fig.1shows these lower masses as a function of orbital radius.Wefind that for planets in the5–30AU range,a planet mass of at least2–5M J masses is required.Thus,it is conceivable that giant planets in the mass range2to 5M J clear the gaps and still remain undetected by our survey.However,we point out that in the cases of most of our targets,and in particular for HD35850,the parameter space that such a planet can occupy is limited.Given that our high–contrast imaging survey did notfind single,large–separation giant planets that may be responsible for clearing the inner disks,we briefly explore alternative mechanisms.Besla&Wu(2007)provides a useful summary of the proposed models and we will only highlight here a particularly interesting proposal proposed recently byMoro-Martin et al.(2007)for the system HD38529,where two close–in planets trigger secular resonances that affect the planetesimal population in the outer disk.In this model the eccentricity of the planetesimals at the location of the secular resonances is excited, thus enhancing the rate of collisions and truncating the planetesimal disk.Given the range of possible mechanisms that may lead to the formation of dust rings it is probable that in–depth studies of the individual systems will be required for judging the feasibility of the proposed models on a case–by–case basis.However,our non–detections show that the presence of evacuated large inner holes in cold disks could be related to localized dust production or concentration of dust grains by dust–gas interactions rather than dynamical clearing by single massive giant planets.5.ConclusionsWe present results from a high–contrast angular differential imaging survey of8cold debris disks,selected to have significantly or totally evacuated inner disks.Our observations searched for massive giant planets that may be responsible for carving out the inner holes in the observed cold debris disks.For most of our targets we reach typical sensitivities of3 to7M J between20to50AU separations,but did not identify any likely planet or brown dwarf candidates.By comparing the derived planet mass upper limits to lower limits derived from dynamical scattering models(typically2-5M J between10and30AU),we limit the parameter space available for any single planet capable of efficiently clearing out the inner planetesimal disks.Our survey complements recent direct imaging surveys of nearby young stars indicating that massive giant planets at large separations are very rare.Cool debris disks with largeinner evacuated cavities remained promising possible exceptions to this rule until now. However,the combination of our observational upper limits and theoretical lower limits strongly suggest that massive giant planets at large separations are not present in most of these systems,reinforcing thefinding that the outer cut–offfor the giant planet distribution is probably at15AU or at even smaller semi–major axes(Kasper et al.2007).We thank the staffat the Paranal Observatories for the support of the service mode observations.In particular,we are grateful to S.Mengel and G.Lowell–Tacconi for their help with the preparation of the observations.This material is partly based upon work supported by the National Aeronautics and Space Administration through the NASA Astrobiology Institute under Cooperative Agreement No.CAN-02-OSS-02issued through the Office of Space Science to the Life and Planets Astrobiology Center(LAPLACE).We would like to thank members of the FEPS team for their help in characterizing the target stars and their disks.FEPS is pleased to acknowledge support through NASA contracts 1224768,1224634,and1224566administered through JPL.Facilities:VLT(NACO).REFERENCESArdila,D.R.,Golimowski,D.A.,Krist,J.E.,et al.2004,ApJ,617,L147Baraffe,I.,Chabrier,G.,Barman,T.S.,Allard,F.,&Hauschildt,P.H.2003,A&A,402, 701Barnes,S.A.2007,ArXiv e-prints,704Beichman,C.A.,Bryden,G.,Rieke,G.H.,et al.2005,ApJ,622,1160Besla,G.&Wu,Y.2007,ApJ,655,528Biller,B.A.,Close,L.M.,Masciadri,E.,et al.2007,ArXiv e-prints,705Burrows,A.,Sudarsky,D.,&Lunine,J.I.2003,ApJ,596,587Greaves,J.S.,Holland,W.S.,Wyatt,M.C.,et al.2005,ApJ,619,L187Hollenbach,D.,Gorti,U.,Meyer,M.,et al.2005,ApJ,631,1180Janson,M.,Brandner,W.,Henning,T.,et al.2007,AJ,133,2442Kasper,M.,Apai,D.,Janson,M.,&Brandner,W.2007,ArXiv Astrophysics e-printsKellner,S.A.2005,PhD thesis,PhD Thesis,Combined Faculties for the Natural Sciences and for Mathematics of the University of Heidelberg,Germany.VIII+129pp.(2005)Kim,J.S.,Hines,D.C.,Backman,D.E.,et al.2005,ApJ,632,659Lafreniere,D.,Doyon,R.,Marois,C.,et al.2007,ArXiv e-prints,705Lenzen,R.,Close,L.,Brandner,W.,Biller,B.,&Hartung,M.2004,in Presented at the Society of Photo-Optical Instrumentation Engineers(SPIE)Conference,Vol.5492, Ground-based Instrumentation for Astronomy.Edited by Alan F.M.Moorwoodand Iye Masanori.Proceedings of the SPIE,Volume5492,pp.970-977(2004).,ed.A.F.M.Moorwood&M.Iye,970–977Lenzen,R.,Hartung,M.,Brandner,W.,et al.2003,in Presented at the Society of Photo-Optical Instrumentation Engineers(SPIE)Conference,Vol.4841,Instrument Design and Performance for Optical/Infrared Ground-based Telescopes.Edited by Iye,Masanori;Moorwood,Alan F.M.Proceedings of the SPIE,Volume4841,pp.944-952(2003).,ed.M.Iye&A.F.M.Moorwood,944–952Levison,H.F.,Morbidelli,A.,Gomes,R.,&Backman,D.2007,Protostars and Planets V, 669Luhman,K.L.,Stauffer,J.R.,&Mamajek,E.E.2005,ApJ,628,L69Mamajek,E.E.,Meyer,M.R.,Hinz,P.M.,et al.2004,ApJ,612,496Marley,M.S.,Fortney,J.J.,Hubickyj,O.,Bodenheimer,P.,&Lissauer,J.J.2007,ApJ, 655,541Marois,C.,Lafreni`e re,D.,Doyon,R.,Macintosh,B.,&Nadeau,D.2006,ApJ,641,556Masciadri,E.,Mundt,R.,Henning,T.,Alvarez,C.,&Barrado y Navascu´e s,D.2005,ApJ, 625,1004Meyer,M.R.,Backman,D.E.,Weinberger,A.J.,&Wyatt,M.C.2007,in Protostars and Planets V,ed.B.Reipurth,D.Jewitt,&K.Keil,573–588Meyer,M.R.,Hillenbrand,L.A.,Backman,D.,et al.2006,PASP,118,1690Meyer,M.R.,Hillenbrand,L.A.,Backman,D.E.,et al.2004,ApJS,154,422Morbidelli,A.,Levison,H.F.,&Gomes,R.2007,ArXiv Astrophysics e-printsMoro-Mart´ın,A.,Carpenter,J.M.,Meyer,M.R.,et al.2007,ApJ,658,1312Moro-Mart´ın,A.&Malhotra,R.2005,ApJ,633,1150Moro-Martin,A.,Malhotra,R.,Carpenter,J.M.,et al.2007,ArXiv e-prints,706Mueller,M.&Weigelt,G.1987,A&A,175,312Pascucci,I.,Gorti,U.,Hollenbach,D.,et al.2006,ApJ,651,1177Quillen,A.C.2006,MNRAS,372,L14Rousset,G.,Lacombe,F.,Puget,P.,et al.2003,in Presented at the Society of Photo-Optical Instrumentation Engineers(SPIE)Conference,Vol.4839,Adaptive Optical System Technologies II.Edited by Wizinowich,Peter L.;Bonaccini,Domenico.Proceedings of the SPIE,Volume4839,pp.140-149(2003).,ed.P.L.Wizinowich&D.Bonaccini, 140–149Setiawan,J.,Weise,P.,Henning,T.,et al.2007,ApJ,660,L145Song,I.,Zuckerman,B.,&Bessell,M.S.2003,ApJ,599,342Takeuchi,T.&Artymowicz,P.2001,ApJ,557,990Williams,J.P.,Najita,J.,Liu,M.C.,et al.2004,ApJ,604,414Wilner,D.J.,Holman,M.J.,Kuchner,M.J.,&Ho,P.T.P.2002,ApJ,569,L115Wyatt,M.C.2005,A&A,433,1007Zuckerman,B.,Song,I.,&Bessell,M.S.2004,ApJ,613,L65。
英语 人类的故事 从宇宙到地球

The birth of nebulae and stars
Gravity and form particle swarm on each particle, which attract more particles, gas ball under appropriate conditions, in the center of the hot, dense enough, can generate burning helium and hydrogen producing stars. Born in the right as the stars in the Eagle nebula.
The formation and early history of the earth
4.6 billion years ago, the primitive earth, dead frozen world, through three effect to gain energy and began to evolution and development. • 1 ° impact effect: interstellar dust high-speed collision, the kinetic energy to heat energy • 2 ° compression effect: interstellar dust landing on the surface, increase external quality, internal squeezed, compression can be converted into heat energy. • 3 ° radioactive decay effect: radioactive elements in the interior of the earth (uranium, thorium, rubidium, etc.). Release huge energy • Evolution results: composition of the molten iron flows to the center of the earth, the formation of iron core, it is a rocky composition form structures (silicate).
宇宙ppt课件英文

Black Hole Types
Different types of black holes including stellar mass black holes, supermassive black holes, and intermediate mass black holes and their characteristics and distribution in the universe.
宇宙ppt课件英文
CATALOGUE
目录
IntroductionThe Structure of the UniverseThe Evolution of the UniverseThe Science of the UniverseThe Applications of the UniverseConclusion
The evolution of stars over time, including the life cycle of stars from birth to death and the different stages of stellar evolution.
Planet Formation
Quasar Properties
The properties of quasars including their luminosity, redshift, and spectral energy distribution.
五年级英语天文现象单选题50题

五年级英语天文现象单选题50题1. There are eight planets in the solar system. Which one is the biggest?A. EarthB. JupiterC. MarsD. Venus答案:B。
本题考查太阳系中行星的大小知识。
地球(Earth)不是最大的行星;火星(Mars)比木星小;金星(Venus)也比木星小。
木星 Jupiter)是太阳系中最大的行星。
2. The sun is a star. What gives the sun its energy?A. WaterB. CoalC. Nuclear fusionD. Wind答案:C。
本题涉及太阳能量来源的知识。
水(Water)、煤炭(Coal)和风(Wind)都不是太阳的能量来源。
太阳的能量来自核聚变(Nuclear fusion)。
3. Which planet is known as the "Red Planet"?A. MercuryB. SaturnC. MarsD. Uranus答案:C。
本题考查对行星特征的了解。
水星(Mercury)不是红色的;土星(Saturn)有环,但不是红色的;天王星(Uranus)也不是红色的。
火星 Mars)表面呈现红色,被称为“红色星球”。
4. How many moons does Saturn have?A. 53B. 62C. 82D. 100答案:C。
本题考查土星卫星数量的常识。
土星有82 颗卫星,A 选项53 颗、B 选项62 颗、D 选项100 颗均不符合实际情况。
5. Which is the closest planet to the sun?A. MercuryB. VenusC. EarthD. Mars答案:A。
本题考查太阳系行星与太阳的距离。
金星 Venus)、地球 Earth)、火星 Mars)距离太阳都比水星远,水星 Mercury)是距离太阳最近的行星。
上海市浦东新区2021届高三上学期一模英语试题含解析
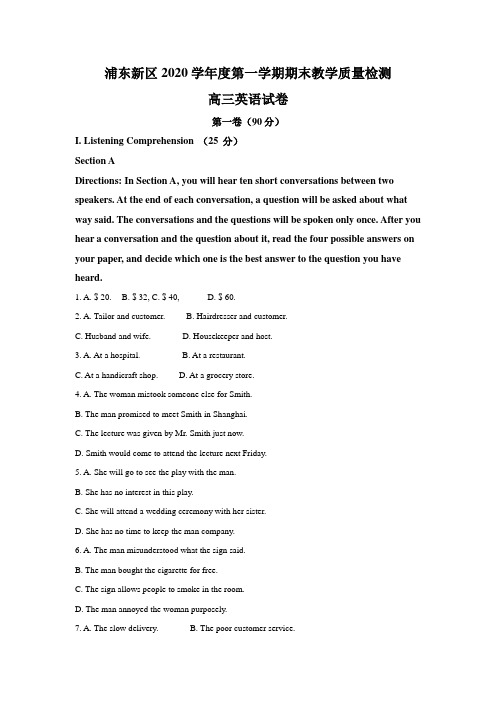
18. A. His flight number and arrival time.
B. The cause of the flight's late arrival.
C. The number of his luggage check.
D. It can create smells and give them off to any scene.
15. A. It helps shoppers locate the right brand of perfume.
B. It helps shoppers check out the perfumes before they buy.
阅读下面短文,在空白处填入1个适当的单词或括号内单词的正确形式。
Since astronomers confirmed the presence of planets beyond our solar system, called exoplanets, humans_________1_________(wonder) how many could harbor life.
Our galaxy holds at least an______4______(estimate) 300 million of these potentially habitable worlds, based on even the most conservative interpretation of the results in a new study to be published in The Astronomical Journal.
The formation of galaxy disks in a hierarchical universe
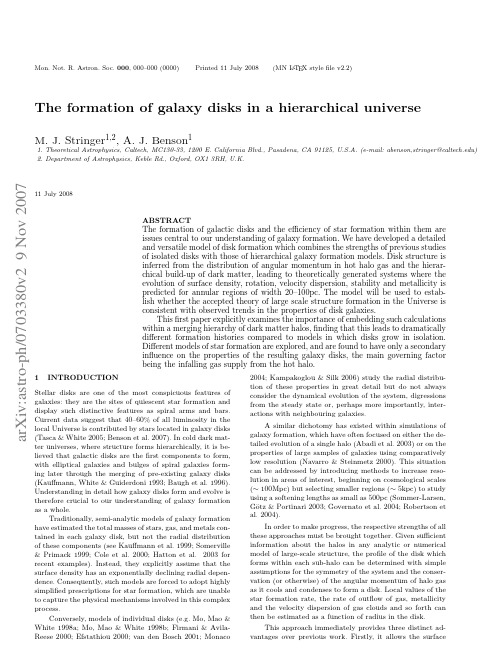
arXiv:astro-ph/0703380v2 9 Nov 2007
11 July 2008
ABSTRACT
The formation of galactic disks and the efficiency of star formation within them are issues central to our understanding of galaxy formation. We have developed a detailed and versatile model of disk formation which combines the strengths of previous studies of isolated disks with those of hierarchical galaxy formation models. Disk structure is inferred from the distribution of angular momentum in hot halo gas and the hierarchical build-up of dark matter, leading to theoretically generated systems where the evolution of surface density, rotation, velocity dispersion, stability and metallicity is predicted for annular regions of width 20–100pc. The model will be used to establish whether the accepted theory of large scale structure formation in the Universe is consistent with observed trends in the properties of disk galaxies. This first paper explicitly examines the importance of embedding such calculations within a merging hierarchy of dark matter halos, finding that this leads to dramatically different formation histories compared to models in which disks grow in isolation. Different models of star formation are explored, and are found to have only a secondary influence on the properties of the resulting galaxy disks, the main governing factor being the infalling gas supply from the hot halo.
7月14日托福阅读真题解析
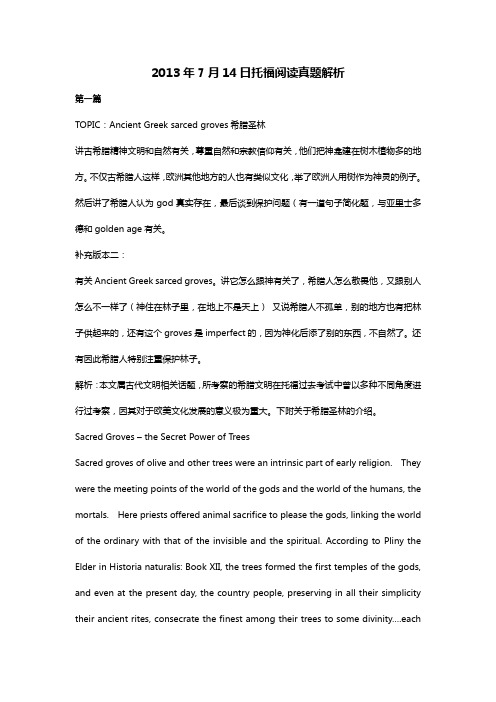
2013年7月14日托福阅读真题解析第一篇TOPIC:Ancient Greek sarced groves希腊圣林讲古希腊精神文明和自然有关,尊重自然和宗教信仰有关,他们把神龛建在树木植物多的地方。
不仅古希腊人这样,欧洲其他地方的人也有类似文化,举了欧洲人用树作为神灵的例子。
然后讲了希腊人认为god真实存在,最后谈到保护问题(有一道句子简化题,与亚里士多德和golden age有关。
补充版本二:有关Ancient Greek sarced groves。
讲它怎么跟神有关了,希腊人怎么敬畏他,又跟别人怎么不一样了(神住在林子里,在地上不是天上)又说希腊人不孤单,别的地方也有把林子供起来的,还有这个groves是imperfect的,因为神化后添了别的东西,不自然了。
还有因此希腊人特别注重保护林子。
解析:本文属古代文明相关话题,所考察的希腊文明在托福过去考试中曾以多种不同角度进行过考察,因其对于欧美文化发展的意义极为重大。
下附关于希腊圣林的介绍。
Sacred Groves – the Secret Power of TreesSacred groves of olive and other trees were an intrinsic part of early religion. They were the meeting points of the world of the gods and the world of the humans, the mortals. Here priests offered animal sacrifice to please the gods, linking the world of the ordinary with that of the invisible and the spiritual. According to Pliny the Elder in Historia naturalis: Book XII, the trees formed the first temples of the gods, and even at the present day, the country people, preserving in all their simplicity their ancient rites, consecrate the finest among their trees to some divinity….eachkind of tree remains immutably consecrated to its own peculiar divinity, the beech to Jupiter, the laurel to Apollo, the olive to Minerva, the myrtle to Venus, and the poplar to Hercules: besides which, it is our belief that the Sylvans, the Fauns, and various kinds of goddess Nymphs, have the tutelage of the woods, and we look upon those deities as especially appointed to preside over them by the will of heaven.The ancient Greeks and the Romans were not the only people to hold the tree sacred. Sacred groves were, and can still be found scattered as far afield as India, the Middle East, Africa, Japan and any region with Celtic ancestry. Celtic sacred groves were called Nemetons, or Nemeta. They were the meeting places of the druids who preferred to perform their rituals in the open air. Many considered them no-go areas where only the initiated could venture safely.The ancient Roman poet Lucan described a sacred grove near modern-day Marseilles like this: no bird nested in the nemeton, nor did any animal lurk nearby; the leaves constantly shivered though no breeze stirred. Altars stood in its midst, and the images of the gods. Every tree was stained with sacrificial blood. the very earth groaned, dead yews revived; unconsumed trees were surrounded with flame, and huge serpents twined round the oaks. The people feared to approach the grove, and even the priest would not walk there at midday or midnight lest he should then meet its divine guardian.第二篇TOPIC 彗星先说comet不好预测,有的周期长有的短,短的可以看见,然后两个图介绍彗星结构(头部有两个部分加tail),然后说彗星在地球周围没有尾巴很小,接近太阳时就被磁场和太阳风搞大了还多了尾巴。
The Total Number of Giant Planets in Debris Disks with Central Clearings
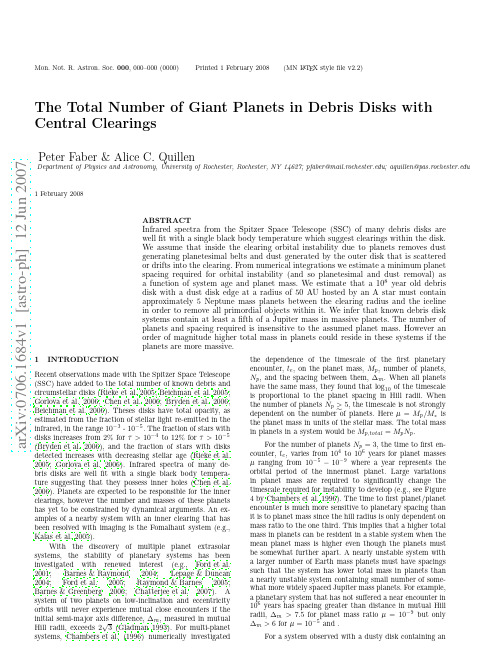
a r X i v :0706.1684v 1 [a s t r o -p h ] 12 J u n 2007Mon.Not.R.Astron.Soc.000,000–000(0000)Printed 1February 2008(MN L A T E X style file v2.2)The Total Number of Giant Planets in Debris Disks withCentral ClearingsPeter Faber &Alice C.QuillenDepartment of Physics and Astronomy,University of Rochester,Rochester,NY 14627;pfaber@;aquillen@ 1February 2008ABSTRACTInfrared spectra from the Spitzer Space Telescope (SSC)of many debris disks are well fit with a single black body temperature which suggest clearings within the disk.We assume that inside the clearing orbital instability due to planets removes dust generating planetesimal belts and dust generated by the outer disk that is scattered or drifts into the clearing.From numerical integrations we estimate a minimum planet spacing required for orbital instability (and so planetesimal and dust removal)as a function of system age and planet mass.We estimate that a 108year old debris disk with a dust disk edge at a radius of 50AU hosted by an A star must contain approximately 5Neptune mass planets between the clearing radius and the iceline in order to remove all primordial objects within it.We infer that known debris disk systems contain at least a fifth of a Jupiter mass in massive planets.The number of planets and spacing required is insensitive to the assumed planet mass.However an order of magnitude higher total mass in planets could reside in these systems if the planets are more massive.1INTRODUCTIONRecent observations made with the Spitzer Space Telescope (SSC)have added to the total number of known debris and circumstellar disks (Rieke et al.2005;Beichman et al.2005;Gorlova et al.2006;Chen et al.2006;Bryden et al.2006;Beichman et al.2006).Theses disks have total opacity,as estimated from the fraction of stellar light re-emitted in the infrared,in the range 10−3-10−5.The fraction of stars with disks increases from 2%for τ>10−4to 12%for τ>10−5(Bryden et al.2006),and the fraction of stars with disks detected increases with decreasing stellar age (Rieke et al.2005;Gorlova et al.2006).Infrared spectra of many de-bris disks are well fit with a single black body tempera-ture suggesting that they possess inner holes (Chen et al.2006).Planets are expected to be responsible for the inner clearings,however the number and masses of these planets has yet to be constrained by dynamical arguments.An ex-amples of a nearby system with an inner clearing that has been resolved with imaging is the Fomalhaut system (e.g.,Kalas et al.2005).With the discovery of multiple planet extrasolar systems,the stability of planetary systems has been investigated with renewed interest (e.g.,Ford et al.2001;Barnes &Raymond 2004;Lepage &Duncan 2004;Ford et al.2005;Raymond &Barnes 2005;Barnes &Greenberg 2006;Chatterjee et al.2007).A system of two planets on low-inclination and eccentricity orbits will never experience mutual close encounters if the initial semi-major axis difference,∆m ,measured in mutual Hill radii,exceeds 2√2Faber&Quilleninterior clearing,we assume that bodies residing between the planets must have been removed via dynamical processes on a timescale shorter than the age of the system.If nu-merous planetesimals lie interior to the clearing they could efficiently produce dust in the clearing.Dust produced in the outer disk can scatter into the clearing because the collision timescales estimated from the dust opacity is significantly shorter than the ages of the systems.Dust can also drift into the clearing because of radiative forces.Studies of dust particle integrations suggest that massive planets,at least Neptune in size,are required to account for a clearing in the dust distribution(e.g.,Ozernoy et al.2000;Liou&Zook 1999;Moro-Mart`ın&Renu2002;Deller&Maddison2005; Quillen2006,2007).Sharp eccentric edges such as that in the Fomalhaut system(Kalas et al.2005)imply that clear-ing by planets is required in some systems(Quillen2006). Our assumption is consistent with scenarios for the evolu-tion of our solar system.Most orbits in between Jupiter and Neptune become planet orbit crossing within the age of the solar system(Duncan et al.1989).Nevertheless cra-tering history suggests that there was an epoch of early bombardment possibly associated with the clearing of inter-planetary debris(e.g.,Gomes et al.2005;Bottke et al.2005; Strom et al.2005).We note that some systems with detected IR excesses may contain evidence for inner exo-zodiacal belts (e.g.,HD12039;Hines et al.2006)in their spectral energy distribution or they may be present but below the detection limit.Nevertheless if planetesimals are originally widely dis-tributed and contain enough mass to form planets(as sug-gested by the cratering record in our solar system),then the lack of bright or detected exo-zodiacal belts implies that most planetesimals have been ejected,accreted onto plane-tary bodies or have fallen into the central star.In this paper we combine the assumptions that planetes-imals within a dust disk edge must be removed within the age of the system with estimates of the timescale t e for the first planet/planet encounter in a multiple planet system. While other explanations for clearing exist,such as a single planet migrating outward constantly eroding away the inner edge of the disk(Gomes et al.2005),we only consider clear-ing via multiple planet induced instability.We estimate a minimum spacing between planets such that objects placed between them are likely to be removed on a timescale shorter than the age of the system while the planets themselves re-main stable.For simulations with planets,only the timescale untilfirst planet/planet encounter depends on the planetary spacing and planet mass.Here we estimate the planet spac-ing for the instability of inter planetary objects by requiring the planets to be twice as far away as required for afirst planet/planet encounter to occur during the age of the sys-tem.Such a system will have planets which will be stable on a timescale much longer than the age of that system. However,particles between the planets will have timescales shorter than the age of the system(e.g.,Duncan et al.1989). We expect that interplanetary particles residing in the sys-tem should have instability timescales similar to those es-timated from N-body simulations of planets spaced at half the spacing.By doubling the spacing,we ensure that un-der these timescales,the planets will be stable while the planetesimals will be cleared.The age of the system is a timescale for planetesimal clearing,not planetary instabil-ity.As all massive bodies influence each other and low mass bodies do not influence massive ones,we expect that our estimate for the required planetary spacing is conservative. Our spacing estimate will be larger than the actual one re-quired for efficient clearing of interplanetary bodies and so will lead to a lower limit on the number of,and total mass, in planets required to clear particles from clearings.2NUMERICAL INTEGRATIONSIntegrations were done using John Chambers’MERCURY package version6.1Mercury6contains several N-body al-gorithms.We use the hybrid symplectic/Burlisch integrator since it is relatively fast and has the ability to compute close encounters.Ten massive bodies of all the same mass ratio were used. Chambers et al.(1996)finds that as long as the number of planets in a system exceedsfive,then the mean stability is nearly independent of number of planets.That is,a system offive planets has similar close encounter timescale depen-dence on separation as a system of ten or twenty planets.As we desire an estimate for the number of planets,we compute the stability timescale for a larger number of bodies in such a way as to be insensitive to actual number of planets.Each body has zero initial eccentricity,inclination,longitude,pe-riapse and mean anomaly.All planets were assumed to have density1g cm−3.They felt no non-gravitational forces.The initial semi-major axis,a n+1,of the n+1-th planet was initially set to depend only on the semi-major axis of the planet just interior or a n with a spacingδsuch thata n+1=(1+δ)a n(1) = 1+∆ r HµMulti-Planet Systems with Debris Disks30 1 2 3 4 5 6 7 00.511.52 2.53 3.5 4l o g (t e )δ/µ(1/4)log(t e ) = A + Blog(µ/10-7) + C(δ/µ(1/4))A = -0.1B = -1.0C = 3.2µ = 10-3µ = 10-5µ = 10-7Figure 1.The relationship between planet spacing,δ,(see equa-tion 2)and time tofirst closeplanet/planetencounter,for systemswith 10coplanar planets.The spacings are multiplied by µ1/4so that the sets of integrations with the same planet mass lie on lines with the same slope.Three sets of integrations are shown,those with planet mass ratio µ=10−3(pluses),10−5(x’s)and 10−7(open squares).A fit to the three sets of integrations is shown with the solid lines.The fit is described with a single equation that relates timescale of first encounter to planet mass ratio and spacing (equation 2).Figure 1.Chambers et al.(1996)found a linear relationship between timescale of first encounter and planetary spacing in Hill radii but the slope of this relation is dependent on planet mass ratio.Only when rescaled by µ1/4(see their fig-ure 4)does the slope become independent of mass ratio.We confirm this here.We have fit the points shown in Figure 1measured from our integrations with a single equation;log 10(t e3.2log 10(t eR in,(4)where R out is the semi-major axis of the outermost planet and R in is the semi-major axis of the innermost planet.The factor of two is used to ensure that over this timescale,the planetesimals are cleared while the planets themselves remain stable.Equation 4for N p can be solved given R in ,R out ,and as δdepends on t e and µ,given the age of the star and an assumed planet mass ratio.The outermost planet must reside within the dust disk clearing so we can set R out to the radius corresponding to the dust temperature estimated from infrared spectra.We conservatively estimate R in to be the distance at which wa-ter freezes (the iceline)based on the luminosity of the central star and given by:R in =L ∗log 10(1+2δ).(6)The factor of 2for δon the right hand side follows because we have set the planets to be twice as far away as that required for instability in the pure 10-body system (see dis-cussion in section 1).The remaining quantity that we must choose to es-timate the number of planets is the planet mass ratio,µ.Recent studies suggest that maintenance of a clear-ing in the dust distribution requires at least a Nep-tune mass planet (e.g.,Ozernoy et al.2000;Liou &Zook 1999;Moro-Mart`ın &Renu 2002;Deller &Maddison 2005;Quillen 2006,2007).We adopt this mass ratio,µ=5×10−5as a starting point and then will consider the effect of varying this ratio.Once we estimate the number of planets present,the total mass in planets M p,total µM ∗N p .(7)The actual mass in planets is likely to be higher than the above estimate for a number of reasons:There may be mas-sive planets within the iceline.We have assumed that all planets have the same mass.If more massive planets are present then a larger total planet mass could be present.This follows because δis not strongly dependent on planet mass.Our integrations were run with ten planets but we may estimate that the number of required planets is fewer and systems with fewer planets are likely to be more stable.We apply our framework for estimating the minimum number and total mass in planets to the disks studied by Chen et al.(2006).Chen et al.(2006)has measured the radii of the clearings in the dust based on dust temperatures4Faber&Quillenmeasured from spectra observed with the Infrared Spectro-graph on board the SSC.Their sample consists of main se-quence stars which are located within150pc and stars that are part of OB associations or moving groups.The proper-ties of these stars are listed in Table1with our calculated limits on the number of planets,N p,and total mass in plan-ets,M p,total within the clearings.Wefind that3-6planets of Neptune mass ratio are required and the total mass in planets is likely to be larger than∼0.2M J where M J is a Jupiter mass.Our estimates for the number of planets and total mass in planets depends on the assumed planet mass ratio,µ.We investigate how the estimates depend on the assumed ratio.From the same stellar sample we have computed the mean number of planets usingµranging from10−3to10−7.The results are plotted in Figure2.The vertical line on this plot shows the location for Neptune’s mass ratio.Wefind that the total number of planets is insensitive to the assumed mass ratio forµ>10−5.Planets with ratios lower than this are unlikely to be able to effectively eject material from central clearings within the lifetime of the system.While the total number of estimated planets is likely to be low,the total mass in planets could be an order of magnitude higher than given in Table1.Jupiter mass planets with similar number and only slightly larger spacings would be capable ejecting material from the clearings during the lifetime of these systems.It is interesting to compute the total number of plan-ets for our solar system assuming a dust belt in the Kuiper Belt were detected(as predicted by Liou&Zook1999).Us-ing R out=30AU and and an iceline at1.0AU for R in we would predict N p=6.6Neptune mass planets,giving a total planetary mass of0.36M J.Much lower than the ac-tual planetary mass in our solar system in this semi-major axis range(1.4M J),we reiterate that our caclulations rep-resent an absolute lower limit on the total planetary mass of a system.Our solar system easily increases its planetary mass since the planets closer than Neptune have varying mass ratios larger than that of Neptune.Since stabilty is less dependent on mass ratio than spacing,fewer more mas-sive planets exist,thus increasing total planetary mass in the solar system.4CONCLUSIONS AND DISCUSSIONIn this paper we have made the assumption that bodies,both planetesimal and dust particles,within a dust disk clearing must be removed from the clearing within the age of the system via gravitational interactions with planets.This as-sumption is consistent with some scenarios for the early evo-lution of our solar system.This assumption is combined with a rough estimate for the timescale for instability in multi-ple planet systems to estimate the planet spacing required for instability.The result is an estimate for the number of planets likely to reside in debris disk systems that host disks with clearings.Wefind that the number of required planets is3-6,between the dust disk clearing radius and the iceline, for the sample of disks studied by(Chen et al.2006),assum-ing a planet mass ratio like that of Neptune.Wefind that the number of estimated planets is only weakly dependent on the assumed planet mass.At least0.2M J total mass51015201e-071e-061e-050.00010.001AverageNumberofPlanetsµFigure2.We consider the average number of planets for the systems in Table1for assumed mass ratios between10−3and10−7.The curved line,∝(log10µ)−0.25,is a bestfit line for the average number of planets in a system based on mass ratio. Wefind that the number of estimated planets is insensitive to the assumed planet mass ratio for planets sufficiently massive toeffectively empty a clearing.If more massive planets are present then the total mass in planets could be an order of magnitude higher than listed in Table1.in planets is required in each system.The planets could be more massive and an order of magnitude more mass in plan-ets could reside in these systems.Our estimate neglects planets that lie within the ice-line but planets could migrate or form closer to the star. The estimate for the planet spacing is based on integra-tion of a10-body system but our estimated planet number falls below10.Further numerical experiments show thatif the number of planets is decreased tofive,a decreaseinδ/µ1/4by ten percent results in the same instability timescale.The estimate for spacing is twice that for insta-bility in a10-body system but really we require an instabil-ity timescale for massless bodies in between massive bodies (e.g.,as studied by Duncan et al.1989).We have assumed that the planet/planet encounter timescale is related to a clearing timescale and this is not necessarily true as small, low mass objects do not feel forces on each other the way that large,massive bodies do.A more sophisticated treat-ment would require integration of a much larger number of particles and low mass as well as planetary mass bodies. We could also examine the effects of resonances on stabil-ity timescales and the phase space of systems with massive planets as was done by Chatterjee et al.(2007).Such an ex-ploration could improve on the crude constraints on multi-planetary systems based on observations of debris disks that we have explored here.Here we have assumed that central clearing of debris and dust is primarily due to scatteringby planets.However collisional cascade models for dust pro-duction in debris disks predict that the dust production rate drops with time and that the centers of disks evolve faster than than their outer parts(e.g.,Dominik&Decin2003).It is possible that future observations and statistical studies may differentiate between collisional cascade evolution and planetary clearing models.Multi-Planet Systems with Debris Disks5 We thank Richard Edgar,Eric Ford,and AlessandroMorbidelli for interesting discussions and correspondence.Support for this work was in part provided by NationalScience Foundation grants AST-0406823&PHY-0552695,the National Aeronautics and Space Administration underGrant No.∼NNG04GM12G issued through the Origins ofSolar Systems Program,and HST-AR-10972to the SpaceTelescope Science Institute.REFERENCESBarnes,R.,&Raymond,S.N.,2004,ApJ,617,569Barnes,R.,&Greenberg,R.2006,ApJ,647,L163Beichman,C.A.,et al.2005,ApJ,622,1160Beichman,C.A.,et al2006,ApJ,652,1674Bottke,William F.,et al2005,Icar,179,63BBryden,G.,Beichman,C.A.,et al.2006,ApJ,636,1098Chambers,J.E.,Wetherill,G.W.,&Boss,A.P.1996,Icarus,119,261Chambers,J.E.1999,MNRAS,304,793Chatterjee,Sourav,et al.2007,arXic:astro-ph/0703166Chen,C.H.,et al.2006,ApJS,166,351Deller,A.T.,&Maddison,S.T.2005,ApJ,625,398Dominik,C.,&Decin,G.2003,ApJ,598,626deZeeuw,P.T.,Hoogerwerf,R.,de Bruijne,J.H.J.,Brown,A.G.A.,&Blaauw,A.1999,AJ117,354Duncan,M.,Quinn,T.,&Tremaine,S.1989,Icarus,82,402Ford,E.B.,Lystad,V.,&Rasio,F.A.2005,Nature,434,873Ford,E.B.,Havlickova,M.,&Rasio,F.A.2001,Icarus,150,303Gladman,B.1993,Icarus,106,247Origin of the cataclysmic Late Heavy Bombardment pe-riod of the terrestrial planetsGomes,R.,Levison,H.F.,Tsiganis,K.,&Morbidelli,A.2005,Nature,435,466Gorlova,N.,Rieke,G.H.,Muzerolle,J.,Stauffer,J.R.,Siegler,N.,Young,E.T.,&Stansberry,J.H.2006,ApJ,649,1028Hines,D.et al.2006,ApJ,638,1070Kalas,P.,Graham,J.R.,&Clampin,M.2005,Nature,435,1067Lepage,I.,&Duncan,M.J.2004,AJ,127,1755Liou,J.-C.,&Zook,H.A.1999,AJ,118,580Moro-Mart`ın,A.,&Malhotra,R.2002,AJ,124,2305MOzernoy,L.M.,Gorkavyi,N.N.,Mather,J. C.,&Taidakova,T.A.2000,ApJ,537,L147Quillen,A.C.2006,MNRAS,372,L14Quillen,A.C.2007,MNRAS,377,1287Raymond,S.N.,&Barnes,R.2005,ApJ,619,549Rieke,et al.2005,ApJ,620,1010Strom,Robert G.,et al.2005,Sci,309,1847SZuckerman,B.,&Song,I.2004,ApJ,603,7386Faber&QuillenTable1.Stars with Dusty Disks with ClearingsγCas 4.07216.52500.11 3.20.17HR333 2.534 6.67410.11 3.50.1949Cet 2.222 4.54190.05 3.50.19HR506 1.221 1.35 1.70.3 5.80.31γTri 2.6317.65540.17 3.00.16τ3Eri 2.012 3.75130.5 2.40.13HR1082 1.952 3.45110.15 5.90.31HR1570 2.242 4.88220.23 4.60.24ηLep 2.310 5.70300.18 1.20.06HD531430.840.720.480.97a 3.50.19HR3314 2.527 6.83430.19 2.90.16HD95086 1.730 2.857.50.016b 5.50.29βUMa 2.7539.32800.34 3.60.19βLeo 2.019 3.90140.05 3.50.19HD110058?20 3.29100.016b 4.20.22γBoo 2.223 4.77210.27 3.30.18αCrB 2.7339.61850.30 2.60.14HD139664 2.715 1.97 3.60.48 4.20.23HD146897 1.517 2.23 4.60.005b 4.90.26HR6297 1.821 3.45110.42 3.80.20HR6486 1.714 2.71 6.80.24 3.50.19HR6532 3.04710.05930.2 3.30.1778Her 2.6237.44510.05 2.50.13γOph 2.527 6.50390.19 3.00.16HR6670 1.513 2.11 4.1 1.6 3.60.19HD181327 1.920 1.64 2.5 1.4 5.00.27HD191089 1.814 1.83 3.1 1.6 4.10.22HR8799 1.68 2.57 6.10.59 2.30.13SUN 1.030 1.04 1.0 4.6 6.60.36。
Module+5+The+Secret+of+the+Gas+Giant+课件-外研版高中英语选修八
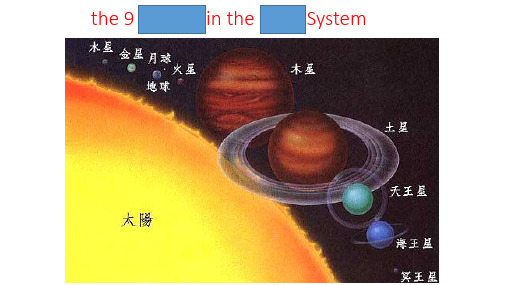
Secrets of the Gas Giant The cassini-Huygens space probe, which reached Saturn last week, has sent back amazing photographs of the planet’s famous rings viewed in ultraviolet light. The pictures showed them in shades of blue, green and red. The different colour show exactly what the rings are made of: the red means the ring contains tiny pieces of rock and the blue and green are likely to be a mixture of water and frozen gases. Saturn itself is made of gases. It is so light that it can float on water-after a big enough ocean could be found! The probe is a international project to explore the planet and their rings and moons. It was launched in 1997 and its mission was to explore the “gas giant” planet where is the furthest planet to be seen from the Earth without a telescope. The probe has sent back pictures of some of Saturn's moons, including tiny Phoebe, which has a strange shape-like other planets and their moons, it is not perfectly round- and Saturn’s biggest moon, Titan, which is believed to be the only body in the solar system rather than the Earth with liquid on the surface.
The solar system
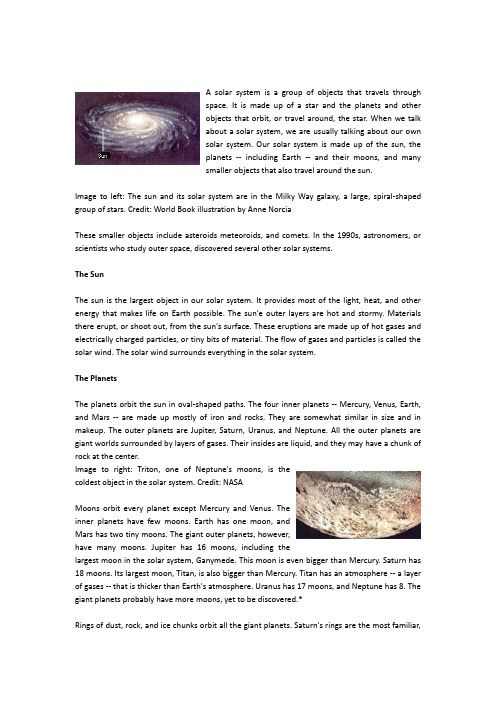
A solar system is a group of objects that travels throughspace. It is made up of a star and the planets and otherobjects that orbit, or travel around, the star. When we talkabout a solar system, we are usually talking about our ownsolar system. Our solar system is made up of the sun, theplanets -- including Earth -- and their moons, and manysmaller objects that also travel around the sun.Image to left: The sun and its solar system are in the Milky Way galaxy, a large, spiral-shaped group of stars. Credit: World Book illustration by Anne NorciaThese smaller objects include asteroids meteoroids, and comets. In the 1990s, astronomers, or scientists who study outer space, discovered several other solar systems.The SunThe sun is the largest object in our solar system. It provides most of the light, heat, and other energy that makes life on Earth possible. The sun'e outer layers are hot and stormy. Materials there erupt, or shoot out, from the sun's surface. These eruptions are made up of hot gases and electrically charged particles, or tiny bits of material. The flow of gases and particles is called the solar wind. The solar wind surrounds everything in the solar system.The PlanetsThe planets orbit the sun in oval-shaped paths. The four inner planets -- Mercury, Venus, Earth, and Mars -- are made up mostly of iron and rocks. They are somewhat similar in size and in makeup. The outer planets are Jupiter, Saturn, Uranus, and Neptune. All the outer planets are giant worlds surrounded by layers of gases. Their insides are liquid, and they may have a chunk of rock at the center.Image to right: Triton, one of Neptune's moons, is thecoldest object in the solar system. Credit: NASAMoons orbit every planet except Mercury and Venus. Theinner planets have few moons. Earth has one moon, andMars has two tiny moons. The giant outer planets, however,have many moons. Jupiter has 16 moons, including thelargest moon in the solar system, Ganymede. This moon is even bigger than Mercury. Saturn has 18 moons. Its largest moon, Titan, is also bigger than Mercury. Titan has an atmosphere -- a layer of gases -- that is thicker than Earth's atmosphere. Uranus has 17 moons, and Neptune has 8. The giant planets probably have more moons, yet to be discovered.*Rings of dust, rock, and ice chunks orbit all the giant planets. Saturn's rings are the most familiar,but thin rings also surround Jupiter, Uranus, and Neptune.CometsComets are objects made up mainly of ice and rock. When a comet gets close to the sun, some of the ice turns into gas. The gas and bits of dust shoot out of the comet. Sunlight pushes against the gas and dust, and the solar wind carries them outward, forming a long tail. Comets come from two regions at the edges of the solar system. Some comets start in the Oort cloud, a far-off cluster of comets. Others come from the Kuiper belt.AsteroidsAsteroids are very small planets. Some travel in oval-shaped orbits that pass inside the orbit of Earth or even that of Mercury. Others travel on a circular path among the outer planets. Astronomers believe there are more than 50,000 asteroids. Most asteroids orbit the sun between the orbits of Mars and Jupiter in a region called the asteroid belt.MeteoroidsMeteoroids are chunks of metal or rock smallerthan asteroids. When meteoroids fall into Earth'satmosphere, they break up. As that happens, theyform bright streaks of light called meteors. Somemeteoroids reach the ground. These meteoroidsare known as meteorites. Most meteoroids arebroken chunks of asteroids. During the 1990s,astronomers discovered a number of meteoroids that came from Mars and from the moon.Image to left: The solar system probably began as a cloud of gas and bits of rock and metal. Credit: World Book illustration by Rob WoodFormation of the Solar SystemMany scientists believe that our solar systemformed from a giant, rotating cloud of gas and tinypieces of rocks and metals. This cloud is known asthe solar nebula. According to this theory, the solarnebula began to shrink. As the nebula got smaller,it spun faster and flattened into a disk.Image to right: The spinning cloud flattened outinto a disk. Credit: World Book illustration by Rob WoodThe scientists believe that particles within the flattened disk then smashed into one another and stuck together to form asteroid-size objects. Some of these objects joined to form the planets.Others formed moons, asteroids, and comets.Image to left: Most of the cloud pulled together inthe center and formed the sun. Credit: World Bookillustration by Rob WoodMost of the material in the nebula, though, waspulled toward the center and formed the sun.According to the theory, the pressure at the centerbecame great enough to cause the nuclearreactions that power the sun. Eventually, solar eruptions occurred, causing a solar wind. In the inner solar system, the wind was so powerful that it swept away most of the lighter elements. In the outer regions of the solar system, however, the solar wind was much weaker. As a result, much more gas remained on the outer planets. That explains why the inner planets are small, rocky worlds and the outer planets are giant ballsof gas.How to cite this article: To cite this article, WorldBook recommends the following format: "Solarsystem." The World Book Student DiscoveryEncyclopedia. Chicago: World Book, Inc., 2005.Image to right: Some of the rock and metal piecessmashed together. They formed planets, moons and asteroids. Credit: World Book illustration by Rob WoodFind this article at:/worldbook/wbkids/k_solarsystem.htmlThe sun is a huge, glowing ball of gases at the center of thesolar system. The Earth and the other eight planets travelaround the sun. The sun is only one of billions of stars in theuniverse, but it is more important to people than any otherstar. Without the heat and light of the sun, there could beno life on the Earth.Image to left: The sun is the largest object in the solarsystem. It is 109 times larger than the Earth. Credit: WorldBook illustration by Roberta PolfusIf the sun were the size of a skyscraper, the Earth would be the size of a person. The moon would be the size of a medium-size dog standing next to the person. Jupiter, the largest planet, would be the size of a small building.The sun is the star that is closest to the Earth. Scientists study the sun to lean about stars that are much farther away. The surface of the sun is made up of hot gases that give off light and heat.How the Sun Affects the EarthSunlight affects the weather of an area. The temperature of any place on the Earth depends on where the sun is in the sky. Tropical areas near the equator, an invisible line around the middle of the Earth, have a hot climate because the sun shine almost directly overhead at noon. Areas near the North Pole and the South Pole have cold weather because the sun never rises very high there.The sun gives us heat, light, and other kinds ofenergy. All life on Earth -- people, animals, andplants -- depends on this energy from the sun.Plants use sunlight to make their own food. As theymake their food, plants give off a gas called oxygen.People and animals eat the plants and breathe inoxygen. In turn, people and animals breathe outthe gas carbon dioxide. Plants mix carbon dioxidewith energy from sunlight and water from the soilto make more food.Image to right: The surface of the sun is made up ofhot gases that give off light and heat. Credit:NASA/ESA/Solar &Heliospheric ObservatoryHow the Sun Was FormedThroughout space, there are huge clouds of gases and dust. New stars are made when parts ofthe gases and dust join together and form a tight mass. The mass starts to make heat. As the mass of gases and dust gets smaller, the center of the mass gets hotter. Finally, the center becomes so hot that the mass of gases and dust begins to shine as a star.Scientists believe the sun was made from a mass of gases and dust. They think the planets were formed from gases and dust that collected at different distances from the center of the sun.How to cite this article: To cite this article, World Book recommends the following format: "Sun." The World Book Student Discovery Encyclopedia. Chicago: World Book, Inc., 2005.Find this article at:/worldbook/wbkids/k_sun.htmlMercury is the planet in our solar system closest to the sun.Because Mercury is small and near the sun, it is often hardto see from Earth without a telescope. A telescope is a toolthat makes faraway objects seem closer and bigger. Atcertain times of the year, Mercury can be seen just aftersunset. At other times, it can be seen just before sunrise.Image to left: The planet Mercury was first photographed indetail on March 29, 1974, by the U.S. probe Mariner 10.Credit: NASAMercury moves around the sun faster than any other planetdoes. The ancient Romans named it Mercury after thespeedy messenger of the gods in ancient Roman stories.Like the moon, Mercury is covered by a thin layer of minerals. It also has wide, flat areas of land, steep cliffs, and many deep craters, or holes, like those on the moon.The inside of Mercury is probably like that of Earth. Many scientists think the insides of both planets are made up mostly of iron.Mercury is dry, very hot, and has almost no air. The sun is much stronger on Mercury than it is on Earth. Scientists believe there is no life on Mercury.The United States Mariner 10 was the first and only spacecraft to reach Mercury. The spacecraft took pictures of Mercury in 1974 and 1975. These pictures tell us all we know about the surface of the planet.How to cite this article: To cite this article, World Book recommends the following format: "Mercury." The World Book Student Discovery Encyclopedia. Chicago: World Book, Inc., 2005.Find this article at:/worldbook/wbkids/k_mercury.htmlVenus is a planet. It is closer to the sun than anyother planet except Mercury. Venus is known asEarth's "twin" because the two planets are aboutthe same size. No other planet is nearer to Earththan Venus.Image to left: Venus is surrounded by thick acidclouds. Astronomers cannot see its surface evenwith telescopes. This picture was made using radarwaves and photos taken from cameras that landedon Venus. Credit: NASAFrom Earth, Venus looks brighter than any otherplanet or even any star in the sky. At certain times of the year, Venus is the first planet or star that can be seen in the western sky in the evening. At other times, it is the last planet or star that can be seen in the eastern sky in the morning. When Venus is bright, it can be seen even in daylight.Mountains and volcanoes rise over much of the planet, and canyons and craters mark its surface. The plants and animals that live on Earth could not live on Venus. Venus is covered with thick clouds of deadly sulfuric acid. It is also much too hot. The temperature on the surface of Venus is about 860 degrees F (460 degrees C), hotter than most ovens. Scientists do not think anything lives on Venus.Scientists have sent spacecraft to Venus to explore the planet. The first spacecraft to pass near Venus was Mariner 2 in 1962.How to cite this article: To cite this article, World Book recommends the following format: "Venus." The World Book Student Discovery Encyclopedia. Chicago: World Book, Inc., 2005.Find this article at:/worldbook/wbkids/k_venus.htmlEarth is the planet we live on. Earth is a huge sphere, or ball,that goes around the sun in a circle. It is covered with water,rock, and soil and surrounded by air. Animals and plants livealmost everywhere on Earth's surface. They can live on Earthbecause it is just the right distance from the sun. Livingthings need the sun's warmth and light. But if Earth werecloser to the sun, it would be too hot for living things. IfEarth were farther from the sun, it would be too cold foranything to live. Also, Earth has plenty of water. Most livingthings need water.Image to right: Seen from space, Earth is a blue ball coveredwith white clouds. This picture was taken by a satellite far out in space. Credit: NASAEarth in spaceEarth is one of the nine planets that travel through space around the sun. Earth is always moving. It spins like a top, and at the same time it travels around the sun. Human beings use these movements to measure the length of days and years on Earth. One day is the time it takes Earth to spin around once. One year is the time it takes Earth to travel once around the sun. Earth has a ball-shaped moon that travels around it the same way the Earth travels around the sun. Outside EarthEarth is not perfectly round. It is a little bit flattened at the poles. The North Pole is at Earth's top, and the South Pole is at its bottom. Halfway between the poles is an imaginary circle around Earth's center. This circle is called the equator. The equator cuts Earth into two halves called the Northern Hemisphere and the Southern Hemisphere.The air that surrounds Earth is called the atmosphere. An invisible gas called nitrogen makes up most of the atmosphere. Oxygen, the gas that people and animals need to breathe, makes up most of the rest. About 1,000 miles (1,600 kilometers) from Earth, the atmosphere fades away into the emptiness of space.Earth's surface is about 70 percent water. Almost all of this water is in Earth's oceans. These oceans form one great body of water often called the world ocean or global ocean. Land makes up about 30 percent of Earth. The largest pieces of land are called continents. There are seven continents. They are Asia, Africa, Antarctica, Australia, Europe, North America, and South America.Earth is covered with a rocky "skin" called the crust. The crust is about 5 miles (8 kilometers) thick under the oceans, and about 25 miles (40 kilometers) thick under the continents. The crust is made up of about 30 huge pieces, called plates, that fit together like a jigsaw puzzle.Inside EarthUnderneath the crust, Earth has three layers of hotrock and metal. These layers are the mantle, theouter core, and the inner core. The mantle is a layerof hot, melted rock below the crust. It is about1,800 miles (2,900 kilometers) thick. The outer corelies beneath the mantle. It is made mostly ofmelted iron, and it is even hotter than the mantle.The inner core is the hottest part of Earth. Scientistsbelieve that the inner core is a ball of solid iron.Image to left: Earth is made up of four layers -- thecrust, the mantle, the outer core and the inner core.Credit: World Book illustration by Raymond Perlman How Earth beganScientists believe that Earth is at least 4.5 billion years old. They think Earth began as a dry ball of rock covered by a cloud of gas. The rock began to heat up, and the inside of Earth melted. Heavy materials, like iron, sank to Earth's middle and formed the core. The lighter rocks moved to the top and formed the first crust.The heating of the inside of Earth caused other chemicals to rise to the surface. Some of these chemicals formed water. Others formed the gases that make up the atmosphere. Over millions of years, the water slowly collected in low places on the crust and formed the oceans.How Earth changesEarth's surface is always changing. Some of these changes happen suddenly. For example, earthquakes break hillsides apart and cause cracks in the ground. Floods wash away soil and form new lakes and rivers. Volcanoes erupt, or explode, burying huge areas in ash and rock.Other changes happen slowly. For example, water and wind are part of a process called erosion. Erosion takes place when parts of the Earth's surface are worn down and their materials are moved somewhere else. Over millions of years, water and wind can make great changes. For example, erosion caused by flowing water carved the Grand Canyon in North America out of hard rock.Other slow changes happen because Earth's plates are always moving. The plates glide along slowly on a layer of very hot rock. In some places, the plates bump into each other. This creates mountains. In other places, the plates move apart. This causes new crust to form. These changes take millions of years.The drifting plates moved Earth's continents to where they are today. Scientists who study Earth believe that hundreds of millions of years ago there was only one huge continent, which scientists called Pangaea. There was only one ocean, called Panthalassa. About 200 million years ago, the plates that formed Pangaea began to drift apart. Pangaea split into two continents called Gondwanaland and Laurasia. Slowly, Gondwanaland broke apart. It formed the continents of Africa, Antarctica, Australia, and South America. Laurasia split into Eurasia and North America. The movement of the continents to where they are now took place over millions of years.How to cite this article: To cite this article, World Book recommends the following format: "Earth." The World Book Student Discovery Encyclopedia. Chicago: World Book, Inc., 2005.Find this article at:/worldbook/wbkids/k_earth.htmlMars is the fourth planet from the sun. It is a reddishplanet covered with rocks and craters, or big holes.Mars was named after the ancient Roman god ofwar. Some scientists believe life may have existed onMars billions of years ago, but there is no proof thatanything is alive on the planet today.Image to left: The planet Mars, like Earth, has cloudsin its atmosphere and a deposit of ice at its northpole. But unlike Earth, Mars has no liquid water onits surface. The rust-like color of Mars comes fromthe large amount of iron in the planet's soil. Credit:NASA/JPL/Malin Space Science SystemsMars travels around the sun in an elliptical, or oval-shaped, orbit. It takes about 687 Earth days for Mars to go all the way around the sun. Mars has two small moons called Phobos and Deimos.The surface of Mars looks more like Earth's than the surface of any other planet does. Even so, the plants and animals that live on Earth could not live on Mars. Its ground is too cold -- it is usually below 32 degrees Fahrenheit (zero degrees Celsius), the freezing point of water. Also, the air of Mars has almost no oxygen. People and animals need oxygen to breathe.Scientists have studied Mars through telescopes for many years. In 1965, the United States spacecraft Mariner 4 flew near Mars and took pictures of its surface. In 1976, the U.S. Viking 1 and Viking 2 were the first spacecraft to land on Mars. They took pictures and collected soil samples. The U.S. Pathfinder probe landed on Mars in 1997. All these spacecraft were controlled by radio signals from Earth. As yet, no people have set foot on Mars.How to cite this article: To cite this article, World Book recommends the following format: "Mars." The World Book Student Discovery Encyclopedia. Chicago: World Book, Inc., 2005.Find this article at:/worldbook/wbkids/k_mars.htmlJupiter is the fifth planet from the sun and thelargest planet in the solar system. More than 1,000Earths would fit inside Jupiter. When viewed fromEarth, Jupiter appears brighter than most stars.Among the planets, only Venus is brighter. Jupiteris named after the king of the Roman gods.Image to left: Jupiter is a giant ball of gas and liquid. Credit: World Book illustration by Herb HerrickJupiter is a giant ball of gas and liquid. It has little or no solid surface. Instead, the planet's surface is made of thick red, brown, yellow, and white clouds. The clouds have dark- and light-colored areas. These areas circle the planet and give it a striped appearance.Jupiter's most outstanding surface feature is the Great Red Spot, a swirling mass of gas. It looks like a TV weather display of a hurricane. The color of the Great Red Spot varies from brick-red to brownish. Jupiter has three thin rings around its middle. They seem to be made mostly of dust particles.Jupiter rotates, or spins, faster than any other planet. Jupiter's day -- that is, the time it takes to spin around once -- is only about 10 hours long. By comparison, Earth's day is 24 hours long. Jupiter takes about 12 years to travel once around the sun, while Earth takes one year.Jupiter has 63 known moons. These moons revolve around Jupiter the way our moon revolves around Earth. Scientists have discovered volcanoes on the moon called Io. They believe that the moon called Europa contains water.How to cite this article: To cite this article, World Book recommends the following format: "Jupiter." The World Book Student Discovery Encyclopedia. Chicago: World Book, Inc., 2005.Find this article at:/worldbook/wbkids/k_jupiter.htmlSaturn is the second largest planet. Only Jupiter is Array larger. Saturn has seven thin, flat rings around it.The rings are made up of many thin ringlets, orsmall rings. The ringlets are made up of smallpieces of ice. All these shiny pieces travel aroundthe planet, making Saturn one of the mostbeautiful objects in space. Jupiter, Neptune, and Uranus are the only other planets that are known to have rings.Image to left: Saturn, the second largest planet in the solar system, has seven rings made up of shiny bits of ice. In this photograph, a section of the rings is hidden by the planet's shadow. Credit: NASA/JPL/Space Science InstituteSaturn can be seen from Earth. However, the rings of Saturn cannot be seen from Earth without a telescope.Saturn is the sixth closest planet to the sun. It travels around the sun in an oval-shaped orbit, or path. The planet takes about 10,759 Earth days, or about 29.5 Earth years, to go around the sun. Earth takes 365 days, or one year, to travel around the sun.Saturn rotates, or spins around, faster than any other planet except Jupiter. Saturn spins around once in only 10 hours and 39 minutes. Earth rotates once in 24 hours, or one day.Most scientists believe Saturn is a giant ball of gas that has no solid, or hard, surface. However the planet seems to have a hot, solid center of iron and rocky material. Scientists do not think that any form of life exists on Saturn. Saturn has at least 18* satellites, or moons. The largest moon is Titan.How to cite this article: To cite this article, World Book recommends the following format:Find this article at:/worldbook/wbkids/k_saturn.htmlUranus is the seventh planet from the sun. Only Neptune isfarther from the sun. Uranus is the most distant planet wecan see without a telescope.Image to left: Uranus is a giant blue-green planet. Credit:NASAUranus is a giant ball of gas and liquid. It is about four timesthe size of Earth. Scientists believe that the surface ofUranus is made up of blue-green clouds of methane, a gas.Beneath the surface are thick layers of water mixed withanother gas called ammonia. The center of the planet may be a rocky core, just like Earth's. Scientists do not think there is any life on Uranus.Uranus was the first planet discovered since ancient times. William Herschel discovered it in 1781. Herschel was a British astronomer, a scientist who studies planets and stars. Scientists have learned much about Uranus from the flight of the United States spacecraft Voyager 2. The spacecraft flew close to Uranus and took pictures. It sent these pictures back to Earth.Uranus moves around the sun in an oval path. It takes about 84 Earth years for Uranus to go around the sun. (It takes one year for Earth to go around the sun.) Like Earth, Uranus also slowly spins on its axis, a make-believe line through the center of the planet. Uranus takes 17 hours and 14 minutes to spin once all the way around on its axis.How to cite this article: To cite this article, World Book recommends the following format: "Uranus." The World Book Student Discovery Encyclopedia. Chicago: World Book, Inc., 2005.Find this article at:/worldbook/wbkids/k_uranus.htmlNeptune is one of the two planets that cannot be seen fromEarth without a telescope. Pluto is the other. Bright blueclouds cover Neptune's surface. Because these clouds looklike water, the planet was named after the ancient Romangod of the sea, Neptune.Image to left: Bright blue clouds surround Neptune. Theyare made of frozen methane, a gas. Because these cloudslook like water, the planet was named after Neptune, theancient Roman god of the sea. Credit: NASA/JPLScientists believe that Neptune is made up mostly of gases, water, and minerals. Plants and animals that live on Earth could not live on Neptune. It is much too cold, and the air has no oxygen. People and animals need oxygen to breathe.Neptune travels around the sun in an elliptical, or oval-shaped orbit. It takes about 165 years for Neptune to go all the way around the sun. It takes our planet Earth one year to go around the sun. Pluto is the only planet farther from the sun than Neptune. But every 248 years, Pluto moves inside Neptune's orbit for about a 20-year period. During that time, Neptune is the farthest planet from the sun.Neptune is about four times as big as Earth. It has at least 13 moons (natural satellites), and several rings. Only two of the moons, Triton and Nereid, can be seen by telescopes on Earth. Six moons and the rings were discovered in 1989 by the United States spacecraft Voyager 2. It took the first close-up pictures of Neptune, although scientists first saw Neptune through a telescope in 1846. Astronomers using powerful telescopes found the other moons in 2002 and 2003.How to cite this article: To cite this article, World Book recommends the following format: "Neptune." The World Book Student Discovery Encyclopedia. Chicago: World Book, Inc., 2005.Find this article at:/worldbook/wbkids/k_neptune.htmlPluto is a far-off dwarf planet. From its discovery in Array 1930, people considered Pluto to be the ninthplanet of our solar system. However, because of itssmall size and irregular orbit, many astronomersquestioned whether Pluto should be grouped withplanets like Earth and Jupiter. In 2006, this debateled the International Astronomical Union, therecognized authority in naming heavenly objects,to formally classify Pluto as a dwarf planet.Image to right: Pluto is so far from Earth that even powerful telescopes show little detail of its surface. The Hubble Space Telescope took these pictures of Pluto. Credit: NASAPluto's path around the sun takes it farther away from the sun than any other planet. Pluto cannot be seen from Earth without a telescope.Pluto is about 39 times as far from the sun as Earth is. It takes about 248 years for Pluto to make one trip around the sun. For about 20 years during each trip, it comes closer to the sun than Neptune does.Pluto is much smaller than Earth, and it is very cold. Pluto has one moon.*In 1996, the Hubble Space Telescope took the first good pictures of Pluto. The planet has large, bright areas that are probably frozen gases. In 2006, the U.S. National Aeronautics and Space Administration (NASA) launched the New Horizons probe. The probe was expected to fly by Pluto in 2015.How to cite this article: To cite this article, World Book recommends the following format:Find this article at:/worldbook/wbkids/k_pluto.html。
英语天文知识英语30题
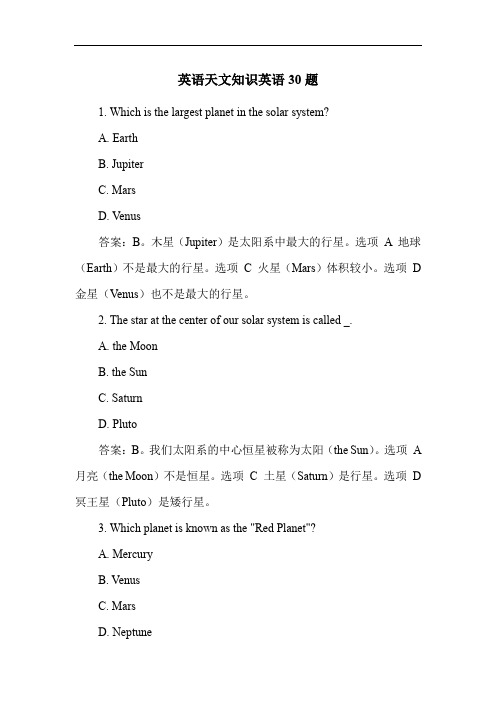
英语天文知识英语30题1. Which is the largest planet in the solar system?A. EarthB. JupiterC. MarsD. Venus答案:B。
木星(Jupiter)是太阳系中最大的行星。
选项A 地球Earth)不是最大的行星。
选项C 火星(Mars)体积较小。
选项D 金星 Venus)也不是最大的行星。
2. The star at the center of our solar system is called _.A. the MoonB. the SunC. SaturnD. Pluto答案:B。
我们太阳系的中心恒星被称为太阳(the Sun)。
选项 A 月亮(the Moon)不是恒星。
选项C 土星(Saturn)是行星。
选项D 冥王星 Pluto)是矮行星。
3. Which planet is known as the "Red Planet"?A. MercuryB. VenusC. MarsD. Neptune答案:C。
火星(Mars)被称为“红色星球”。
选项A 水星(Mercury)不是红色的。
选项B 金星(Venus)不是红色星球。
选项D 海王星Neptune)不是红色的。
4. How many planets are there in the solar system?A. 8B. 9C. 10D. 11答案:A。
太阳系中有8 颗行星。
曾经冥王星被认为是第九颗行星,但现在冥王星被归类为矮行星。
5. Which planet is closest to the Sun?A. MercuryB. VenusC. EarthD. Mars答案:A。
水星(Mercury)是离太阳最近的行星。
选项B 金星Venus)距离太阳比水星远。
选项C 地球(Earth)距离太阳比水星远。
选项D 火星 Mars)距离太阳比水星远。
伽利略之夜 木星 英语版
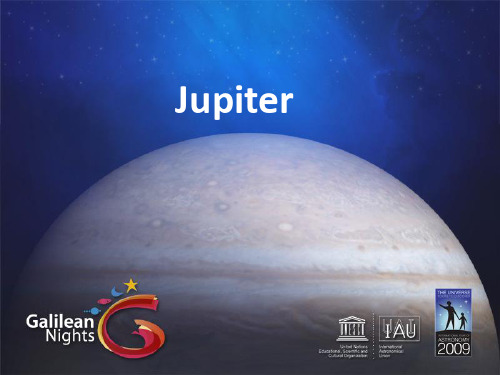
Additional link: Astronomy sketch of the day - /
Other observations
In 1675 Danish astronomer Ole Roemer was able to estimate the speed of light by observing eclipses of the moons Galileo discovered. He deduced that light took longer to travel the extra distance when Earth was farther from Jupiter, compared to when the planets were closer. A few decades later, Isaac Newton used the orbits of the Galilean moons to determine that Jupiter has an enormous mass.
Galileo’s moons: Io
Credit: NASA/JPL/University of Arizona
About the size of Earth’s moon, Io is the closest to Jupiter of the four large moons Galileo discovered. Io is the most volcanically active body in the Solar System, with giant volcanic plumes rising 300 kilometres (190 miles) above the surface. The surface of Io is constantly renewing itself, filling in any impact craters with molten lava lakes and spreading smooth new floodplains of liquid rock.
太阳系的形成
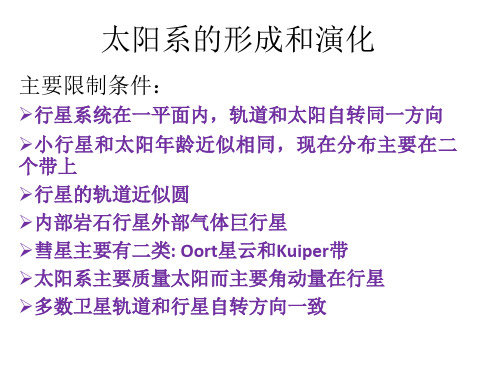
How to form metal/rock-dominated earth-like planets?
当外质停止, 盘冷却,岩石和冰尘埃颗粒沉到吸积盘的中 心—原行星盘(protoplanetary disk)。这些早期尘埃包括在 太阳系中碳质球粒陨石(carbonaceous chondrite meteorites)中 探测到钙铝包体(Calcium-Aluminium Inclusion),包括元素Al, Ca, Ti 和示踪元素Zr, Hf, U, Th, 反射金属W, Ir, Os 等, 形成温度2000K。
22/24
The “Nice” Model
慢迁徙持续~800 Myrs, 木星和土星穿越1:2MMR轨道 二行星的相互作用使得土星的轨道偏心率增大, 从而改 变其他二个行星轨道 在4 Myrs, 行星从原来位置变到现在的位置, planetesimals盘散开,损失97%的质量 小天体被抛向内部(Late Heavy Bombardment), 或者 外部 (Oort Cloud).
The “Nice” Model
R. Gomes, H. F. K.; Gomes, Tsiganis & A. Morbidelli Morbidelli, A.; Levison, H.F.; Tsiganis,Levison, K. R. (2005) Nature, 435 (7041), 462–465 Tsiganis, K.; R. Gomes, A. Morbidelli & H. F. Levison (2005), Nature, 435 (7041): 459–461 R. Gomes, H. F. Levison, K. Tsiganis, A. Morbidelli (2005), Nature 435 (7041): 466
自己设一个行星的英文作文
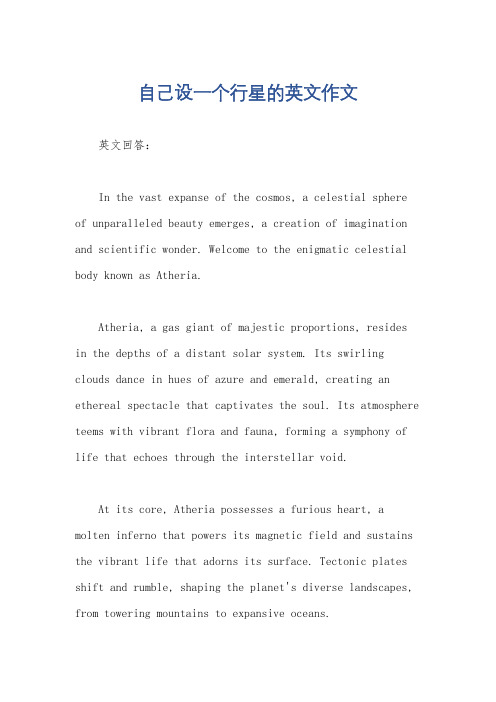
自己设一个行星的英文作文英文回答:In the vast expanse of the cosmos, a celestial sphere of unparalleled beauty emerges, a creation of imagination and scientific wonder. Welcome to the enigmatic celestial body known as Atheria.Atheria, a gas giant of majestic proportions, resides in the depths of a distant solar system. Its swirling clouds dance in hues of azure and emerald, creating an ethereal spectacle that captivates the soul. Its atmosphere teems with vibrant flora and fauna, forming a symphony of life that echoes through the interstellar void.At its core, Atheria possesses a furious heart, a molten inferno that powers its magnetic field and sustains the vibrant life that adorns its surface. Tectonic plates shift and rumble, shaping the planet's diverse landscapes, from towering mountains to expansive oceans.Atheria's orbit takes it on a celestial journey, bringing it into proximity with its neighboring celestial bodies. It engages in a delicate dance with a binary star system, casting intricate shadows upon its clouds. The planet's tilt gives rise to vibrant seasons, each bringing its own unique tapestry of colors and phenomena.As the sun's golden rays bathe Atheria, its surface comes alive with vibrant plant life. Lush forests carpet the land, their verdant canopies reaching towards the heavens. Exotic flowers bloom in a kaleidoscope of colors, releasing a heady fragrance that permeates the air.Atheria's oceans are teeming with an astonishing diversity of marine life. Gigantic creatures navigate the depths with grace and power, while playful dolphins leap and frolic in the waves. Bioluminescent algae illuminate the night sky, transforming the ocean into a celestial tapestry.The inhabitants of Atheria are a testament to theplanet's boundless creativity. Intelligent beings, with their own unique cultures and histories, have flourished on its surface. They have harnessed the planet's resources, harnessing wind and solar energy to power their civilizations.Atheria's skies are adorned with an array of celestial wonders. Auroras dance in vibrant hues, illuminating the horizon with ethereal light. Meteor showers paint streaks of fire across the starlit canvas. And on clear nights, the Milky Way unfurls its shimmering arms, revealing the boundless expanse of the cosmos.中文回答:艾瑟利亚。
- 1、下载文档前请自行甄别文档内容的完整性,平台不提供额外的编辑、内容补充、找答案等附加服务。
- 2、"仅部分预览"的文档,不可在线预览部分如存在完整性等问题,可反馈申请退款(可完整预览的文档不适用该条件!)。
- 3、如文档侵犯您的权益,请联系客服反馈,我们会尽快为您处理(人工客服工作时间:9:00-18:30)。
2
Mayer, Wadsley, Quinn & Stadel
in highly irradiated environments like the Orion nebula (Throop et al. 2001), and are a bit too tight even when compared to the typical lifetime of disks in more quiet environments like Taurus (Haisch, Lada & Lada 2001). One of the strong points of the core accretion model, namely the prediction that gas giants have solid cores, is considerably weakened now that new models of the interior of Jupiter are consistent with the total absence of such a core (Guillot 1999). The discovery of extrasolar planets (Marcy & Butler 1998) has worsened the situation further because now we need to explain the existence of very massive planets, up to ten times larger than Jupiter, that in the standard model would either take too long to be formed, might not form at all (Bate et al. 2003) or could migrate towards the central star before being able to accrete enough mass (Nelson et al. 2000). The distribution of their orbital eccentricities must also be explained; whilst in the past few years several papers have proposed a variety of explanations, sometimes tuned to the properties of one particular system, none of these is valid in general. Mayer et al. (2002, hereafter MA02) showed for the first time that if a massive disk remains cold long enough, until the growing overdensities reach some density threshold, fragmentation takes place even when heating from compression and shocks is included —the rebital times, leading to systems of only a few protoplanets with masses and orbital eccentricities in the range of observed extrasolar planets. The strong point of the 3D smoothed particle hydrodynamics (SPH) simulations of MA02 was the high resolution (up to 106 gas particles) that allowed to resolve the local Jeans length across a wide range of densities, including the regime in which fragmentation takes place (Bate & Burkert 1997; Nelson 2003). However, these simulations where quite simplified in the thermodynamics (see Pickett et al. 2000; 2003), since only two extreme conditions, a locally isothermal or an adiabatic equation of state (with shock heating), were employed. Boss (2001, 2002), by using radiative transfer in the diffusion approximation, has shown that efficient cooling in the disk midplane can occur thanks to convective transport of heat to the disk atmosphere. He finds that the timescale for convective cooling is comparable to the orbital time in the outer, colder regions of a disk of mass ∼ 0.1M⊙. Here we review the main results obtained with the large survey of simulations extensively described in Mayer et al. (2003, hereafter MA03), and we present the first results of new simulations in which radiative cooling and heating are directly implemented. Following recent work by Pickett et al. (2003) and Rice et al. (2003a,b), we will investigate how fast cooling has to be for fragmentation into gravitationally bound planets to occur.
Lucio Mayer
Institute for Theoretical Physics, University of Zu¨rich, CH-8057 Zuric h, Switzerland
James Wadsley
Department of Physics & Astronomy, McMaster University, Canada
arXiv:astro-ph/0311048v2 3 Nov 2003
**TITLE** ASP Conference Series, Vol. **VOLUME***, **YEAR OF PUBLICATION** **NAMES OF EDITORS**
Giant planet formation from disk instability; cooling and heating
Thomas Quinn
Department of Astronomy, University of Washington, Seattle (USA)
Joachim Stadel Institute for Theoretical Physics, University of Zu¨rich, CH-8057 Zuric h, Switzerland
Abstract. We present the results of high resolution SPH simulations of the
evolution of gravitationally unstable protoplanetary disks. We report on calculations in which the disk is evolved using a locally isothermal or adiabatic equation of state (with shock heating), and also on new simulations in which cooling and heating by radiation are explicitly modeled. We find that disks with a minimum Toomre parameter < 1.4 fragment into several gravitationally bound protoplanets with masses from below to a few Jupiter masses. This is confirmed also in runs where the disk is given a quiet start, growing gradually in mass over several orbital times. A cooling time comparable to the orbital time is needed to achieve fragmentation, for disk masses in the range 0.08 − 0.1M⊙. After about 30 orbital times, merging between the bound condensations always leads to 2-3 protoplanets on quite eccentric orbits.