Probing bulk properties and partonic collectivity via multi-strange baryons in Au+Au collis
超特克HV9910BDB7高亮LED驱动示例板说明书

Supertex inc.HV9910BDB7HV9910B PFC 40W LED Driver DemoboardSpecificationsActual Size: 3.0” x 2.3” x 1.1”Top View Bottom ViewGeneral DescriptionThe Supertex HV9910BDB7 demonstrates the use of an HV9910B control IC in an off-line, High Brightness LED driver application. The board incorporates power factor correction (PFC) and satisfies the limits for harmonic currents according to the EN61000-3-2 Class C standard having total harmonic distortion (THD) less than 20%. The board features a low component count and long life operation due to the absence of electrolytic capacitors. The board is designed to supply a string of LEDs with a current of 350mA and a voltage in the 65 to 105V range from a 220/230VAC line.The conversion stage draws line current throughout the AC line cycle, partly using a charge pumping and partly using a boost conversion technique to charge the bulk energy storage capacitors. The LED current is provided with a continuous mode buck stage giving a DC current with about 30% peak-to-peak ripple. A patent for this conversion technique is pending. Please inquire with the Supertex applications department for design guidance, should change of input line voltage, output voltage, or output current be desired.An effort was made to satisfy the requirements of CISPR 15 (EN55015), limits and methods of measurement of radio disturbance characteristics of electrical lighting and similar equipment.The connection diagram details the hookup of the board to the AC line. Note that the load is NOT galvanically isolated, and that measurements to the board require measurement techniques in common use with non-isolated off-line power supplies (isolation transformers, differential probes, etc).Board LayoutSchematic DiagramConnection Diagram220VAC105VDC 350mADCConnectionsI nput Voltage - Connect the AC line input voltage to AC VIN as shown.LED String - Connect the LED strings between LED+ and LED- as shown (anode of the string to LED+ and cathode to LED-).Figure 3. Load regulation.Figure 4. AC line regulation.Figure 6. Power Factor vs. AC Line Voltage.Figure 5. Harmonic Distortion vs. AC Line Voltage.ILoad Regulation (V = 230V )0.3480.3520.3540.3560.3580.3620.3640.366V OI Line Regulation (V = 105V)0.3490.3500.3510.3520.3530.3540.3550.3560.3570.358V ACHarmonic Distortion (V = 105V, I = 350mA)10152025303540V ACPower Factor (V = 105V, I = 350mA)V ACTypical CharacteristicsEfficiency (V = 105V, I = 350mA) V ACEfficiency (V = 230V , I = 350mA)V OFigure 1. Efficiency at full load.Figure 2. Efficiency at nominal AC line voltage.Figure 7. EMI Characterization - Conducted Emissions vs CISPR 15 Limits. Figure 8. CT1 Construction Diagram.Supertex inc. does not recommend the use of its products in life support applications, and will not knowingly sell them for use in such applications unless it receives an adequate “product liability indemnification insurance agreement.” Supertex inc. does not assume responsibility for use of devices described, and limits its liability to the replacement of the devices determined defective due to workmanship. No responsibility is assumed for possible omissions and inaccuracies. Circuitry and specifications are subject to change without notice. For the latest product specifications refer to the Supertex inc. (website: http//)©2013 Supertex inc.All rights reserved. Unauthorized use or reproduction is prohibited.Supertex inc.。
高密度阳极铝电解槽电

第 54 卷第 2 期2023 年 2 月中南大学学报(自然科学版)Journal of Central South University (Science and Technology)V ol.54 No.2Feb. 2023高密度阳极铝电解槽电−热场耦合仿真研究魏兴国1,廖成志1,侯文渊1, 2,段鹏1,李贺松1(1. 中南大学 能源科学与工程学院,湖南 长沙,410083;2. 中北大学 能源与动力工程学院,山西 太原,030051)摘要:在铝电解槽中,阳极炭块内存在的气孔会降低炭块的导电和导热性能,并且增加炭渣,降低电流效率,导致炭耗和直流电耗升高。
通过浸渍工艺得到的高密度阳极可以有效地降低炭块的气孔率。
为了探究高密度阳极铝电解槽的电−热场变化和影响,基于ANSYS 软件建立高密度阳极铝电解槽的电−热场耦合计算模型。
研究结果表明:铝电解槽高密度阳极炭块的平均温度上升8.73 ℃,热应力增加,但形变量减小;侧部槽壳的平均温度下降28.59 ℃,热应力和形变量均降低,有利于保持槽膛内形稳定;热场变化主要与阳极炭块物性改变有关;槽电压降低49.16 mV ,主要与炭块物性改变和电解质电阻率降低有关;高密度阳极电流全导通时间缩短3.39 h ,可有效减弱换极产生的负面影响,阳极使用寿命可延长4 d ,炭耗降低10.3 kg/t ;铝电解槽反应能耗占比增加0.62%,电流效率提高1.69%,直流电耗降低270 kW·h/t 。
关键词:铝电解槽;高密度阳极;电−热场;耦合仿真中图分类号:TF821 文献标志码:A 文章编号:1672-7207(2023)02-0744-10Simulation study of electric-thermal field coupling in high-densityanode aluminum electrolyzerWEI Xingguo 1, LIAO Chengzhi 1, HOU Wenyuan 1, 2, DUAN Peng 1, LI Hesong 1(1. School of Energy Science and Engineering, Central South University, Changsha 410083, China;2. School of Energy and Power Engineering, North University of China, Taiyuan 030051, China)Abstract: In aluminum electrolytic cells, porosity in anode carbon blocks can reduce the electrical and thermal conductivity of the blocks and increase carbon slag, reduce current efficiency and lead to higher carbon consumption and DC power consumption. High-density anodes obtained by impregnation process can effectively reduce the porosity of carbon blocks. In order to investigate the electric-thermal field variation and the causes of influence in the high-density anode aluminum electrolyzer, a coupled electric-thermal field calculation model of收稿日期: 2022 −07 −11; 修回日期: 2022 −08 −20基金项目(Foundation item):国家高技术研究发展项目(2010AA065201);中南大学研究生自主探索创新项目(2021zzts0668)(Project(2010AA065201) supported by the National High-Tech Research and Development Program of China; Project (2021zzts0668) supported by the Independent Exploration and Innovation of Graduate Students in Central South University)通信作者:李贺松,博士,教授,博士生导师,从事铝电解研究;E-mail:****************.cnDOI: 10.11817/j.issn.1672-7207.2023.02.032引用格式: 魏兴国, 廖成志, 侯文渊, 等. 高密度阳极铝电解槽电−热场耦合仿真研究[J]. 中南大学学报(自然科学版), 2023, 54(2): 744−753.Citation: WEI Xingguo, LIAO Chengzhi, HOU Wenyuan, et al. Simulation study of electric-thermal field coupling in high-density anode aluminum electrolyzer[J]. Journal of Central South University(Science and Technology), 2023, 54(2): 744−753.第 2 期魏兴国,等:高密度阳极铝电解槽电−热场耦合仿真研究the high-density anode aluminum electrolyzer was established based on ANSYS software. The results show that the average temperature of the anode carbon block increases by 8.73 ℃ when the high-density anode is put on the tank, and the thermal stress increases but the deformation variable decreases. The average temperature of the side shell decreases by 28.59 ℃, and the thermal stress and deformation variable both decrease,which helps to protect the inner shape of the tank chamber stable. The change of the thermal field is mainly related to the change of the physical properties of the anode carbon block. The cell voltage decreases by 49.16 mV which is mainly related to the change of carbon block physical ploperties and the decrease of electrolyte resistivity, respectively. The reduction of 3.39 h in the full conduction time of high-density anode current can effectively reduce the negative effects of electrode change, and the anode service life can be extended by 4 d. The carbon consumption is reduced by 10.3 kg/t. The reaction energy consumption of aluminum electrolyzer is increased by 0.62%, the current efficiency is increased by 1.69%, and the DC power consumption is reduced by 270 kW·h/t.Key words: aluminum electrolyzer; high-density anode; electric-thermal field; coupling simulation作为铝电解槽的核心部件,阳极炭块在反应过程中被不断消耗,其品质直接影响着各项经济技术指标[1]。
Sweden:LPJ-GUESS

Sweden: LPJ-GUESSThe name of the group: Department of Physical Geography and Ecosystem AnalysisName, title and affiliation of Principal investigator: Assoc. Prof. Almut Arneth; Assoc. Prof. Ben Smith Name and affiliation of contact point, incl. detailed address: Almut Arneth & Ben Smith, INES,Sölvegatan12,22362Lund,*****************************.se;*******************.seURL: http://www.nateko.lu.se/INES/Svenska/main.aspPartner institutions: PIK, SMHI/EC-EARTHWhich components:land sfc yesatmospheric chemistry yesProject Description:LPJ-GUESS (Smith et al., 2001; Hickler et al., 2004) is a generalized, process-based model of vegetation dynamics and biogeochemistry designed for regional to global applications. It combines features of the widely used Lund-Potsdam-Jena Dynamic Global Vegetation Model (LPJ-DGVM; Sitch et al., 2003) with those of the General Ecosys-tem Simulator (GUESS; Smith et al., 2001) in a single, flexible modeling framework. The models have identical representations of ecophysiological and biogeochemical processes, including the hydrological cycle updates d e-scribed in Gerten et al. (2004). They differ in the level of detail with which vegetation dynamics and canopy stru c-ture are simulated: simplified, computationally efficient representations are used in the LPJ-DGVM, while in GUESS a "gap-model" approach is used which is particularly suitable for continental to regional simulations. Tepre-sentations of stochastic establishment, individual tree mortality and disturbance events ensure representation of suc-cessional vegetation dynamics which is important for vegetation response to extreme events.LPJ-GUESS models terrestrial carbon and water cycle from days to millennia (Sitch et al. 2003; Koca et al. 2006; Morales et al. 2005, 2007) and has been shown to reproduce the CO2 fertilisation effects seen in FACE sites (Hickler et al. in press). It has been widely applied to assess impacts on carbon cycle and veg etation based on scenarios from climate models (Gritti et al. 2006; Koca et al. 2006; Morales et al. 2007; Olesen et al. 2007). In addition it has several unique features that are currently not available in any of the Earth System Models:(1) A process-based description for the main biogenic volatile organic compounds (BVOC) emitted by vegetation. BVOC are crucial for air chemistry and climate models, since they contribute to formation and destruction of trop o-spheric O3 (depending on presence and absence of NOx), constrain the atmospheric lifetime of methane, and are key precursors to secondary organic aerosol formation. LPJ-GUESS is the only land surface model with a mechanistic BVOC representation that links their production to photosynthesis. It also uniquely accounts for the recently disco v-ered direct CO2-BVOC inhibition which has been shown to fundamentally alter future and past emissions compared to empirical BVOC algorithms that neglect this effect (Arneth et al., 2007a,b). (2) The possibility to simulate past and present vegetation description on a tree species (as well as PFT) level (Miller et al., in press, Hickler et al., 2004). This is crucial for simulations of BVOC and other reactive trace gases and allows for a much better represe ntation of vegetation heterogeneity in regional and continental atmospheric chemistry-climate studies (Arneth et al., 2007b), an important aspect since spatial heterogeneity must be accounted for with atmospherically reactive chemical species. (3) LPJ-GUESS accounts for deforestation by early human agriculture throughout the Holocene and the effects on global carbon cycle and atmospheric CO2 concentration (Olofsson & Hickler 2007). We currently investigate the impor-tance of Holocene human deforestation on BVOC and fire trace gas and aerosol emissions, and how these may affect Holocene CH4 levels, and simulations of pre-industrial O3. (4) LPJ-GUESS accounts for deforestation by early human agriculture throughout the Holocene and the effects on global carbon cycle and atmospheric CO2 concentra-tion (Olofsson & Hickler 2007). We currently investigate the importance of Holocene human deforestation on BVOC and fire trace gas and aerosol emissions, and how these may affect Holocene CH4 levels, and simulations of pre-industrial O3. (5) A novel global process-based fire description, SPITFIRE (Thonicke et al., 2007) has been incorporated; it is currently used to study effects of climate change and of human vs. natural ignition on carbon cycle and trace gas emissions in savanna ecosystems. (6) Prognostic schemes for agricultural and forest land use that p a-rameterise farmer and forestmanagement decisions under changing climate and productivity. The agricultural scheme has been implemented and applied at the global scale (Bondeau et al. 2007), the forest management scheme in a prototype form for Sweden (Koca et al. 2006). (7) Incorporation of a permafrost module, wetland processes and methane emissions, as well as vegetation nitrogen cycle is in progress.The vegetation dynamics module of LPJ-GUESS has been coupled to the land surface scheme of the Rossby Centre regional climate model RCA3 (Jones et al. 2004a,b) and is being applied to investigate biophysical feedbacks of land surface changes on climate at the regional scale in Europe. The above listed process descriptions are also applicable and available to global ESMs.(References available on request)。
二叠纪-三叠纪灭绝事件
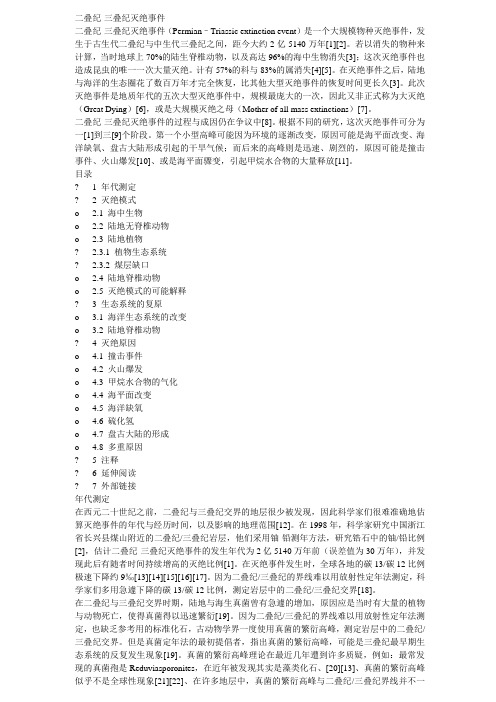
二叠纪-三叠纪灭绝事件二叠纪-三叠纪灭绝事件(Permian–Triassic extinction event)是一个大规模物种灭绝事件,发生于古生代二叠纪与中生代三叠纪之间,距今大约2亿5140万年[1][2]。
若以消失的物种来计算,当时地球上70%的陆生脊椎动物,以及高达96%的海中生物消失[3];这次灭绝事件也造成昆虫的唯一一次大量灭绝。
计有57%的科与83%的属消失[4][5]。
在灭绝事件之后,陆地与海洋的生态圈花了数百万年才完全恢复,比其他大型灭绝事件的恢复时间更长久[3]。
此次灭绝事件是地质年代的五次大型灭绝事件中,规模最庞大的一次,因此又非正式称为大灭绝(Great Dying)[6],或是大规模灭绝之母(Mother of all mass extinctions)[7]。
二叠纪-三叠纪灭绝事件的过程与成因仍在争议中[8]。
根据不同的研究,这次灭绝事件可分为一[1]到三[9]个阶段。
第一个小型高峰可能因为环境的逐渐改变,原因可能是海平面改变、海洋缺氧、盘古大陆形成引起的干旱气候;而后来的高峰则是迅速、剧烈的,原因可能是撞击事件、火山爆发[10]、或是海平面骤变,引起甲烷水合物的大量释放[11]。
目录? 1 年代测定? 2 灭绝模式o 2.1 海中生物o 2.2 陆地无脊椎动物o 2.3 陆地植物? 2.3.1 植物生态系统? 2.3.2 煤层缺口o 2.4 陆地脊椎动物o 2.5 灭绝模式的可能解释? 3 生态系统的复原o 3.1 海洋生态系统的改变o 3.2 陆地脊椎动物? 4 灭绝原因o 4.1 撞击事件o 4.2 火山爆发o 4.3 甲烷水合物的气化o 4.4 海平面改变o 4.5 海洋缺氧o 4.6 硫化氢o 4.7 盘古大陆的形成o 4.8 多重原因? 5 注释? 6 延伸阅读? 7 外部链接年代测定在西元二十世纪之前,二叠纪与三叠纪交界的地层很少被发现,因此科学家们很难准确地估算灭绝事件的年代与经历时间,以及影响的地理范围[12]。
胞外聚合物的提取_组成及其对污泥性质的影响
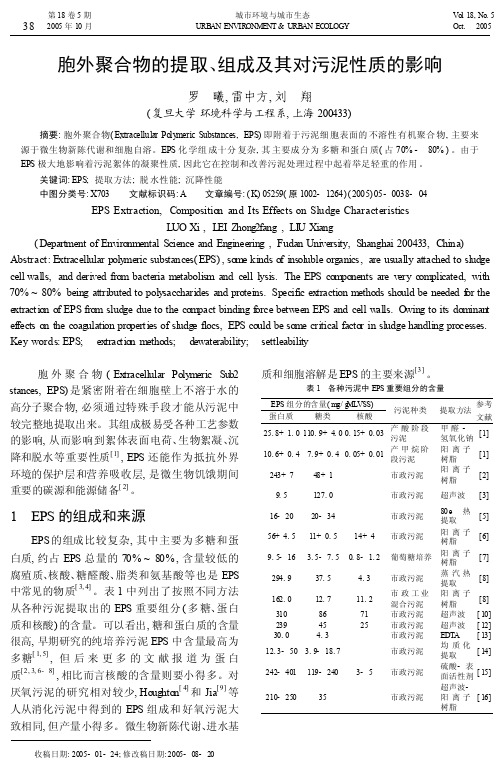
胞外聚合物的提取、组成及其对污泥性质的影响罗曦,雷中方,刘翔(复旦大学环境科学与工程系,上海200433)摘要:胞外聚合物(Extracellular Po ly meric Substances,EPS)即附着于污泥细胞表面的不溶性有机聚合物,主要来源于微生物新陈代谢和细胞自溶。
EPS化学组成十分复杂,其主要成分为多糖和蛋白质(占70%-80%)。
由于EPS极大地影响着污泥絮体的凝聚性质,因此它在控制和改善污泥处理过程中起着举足轻重的作用。
关键词:EPS;提取方法;脱水性能;沉降性能中图分类号:X703文献标识码:A文章编号:(K)05259(原1002-1264)(2005)05-0038-04EPS Extraction,Composition and Its Effects on Sludge CharacteristicsL UO Xi,L EI Z hong2fang,LI U Xiang(Department of Environmental Science and Engineering,Fudan University,Shanghai200433,China) Abstract:E xtracellular polymeric substances(EPS),some kinds of insoluble organics,are usually attached to sludge cell walls,and derived from bacteria metabolism and cell lysis.The EPS components are very c omplicated,with 70%~80%being attributed to polysaccharides and proteins.Specific extraction methods should be needed f or the e xtraction of EPS from sludge due to the compact binding f orce betw een EPS and cell w alls.O wing to its dominant ef fects on the coagulation properties of sludge flocs,EPS could be some critical factor in sludge handling processes. Key words:EPS;extrac tion methods;dewaterability;settleability胞外聚合物(Extracellular Polymeric Sub2 stances,EPS)是紧密附着在细胞壁上不溶于水的高分子聚合物,必须通过特殊手段才能从污泥中较完整地提取出来。
Copy of Echinoderm word

5
Feeding behaviors
• Some echinoderms are ____________, others are detritus foragers (sea cucumbers) or plank tonic feeders (basket stars). • Sea stars are carnivorous and prey on worms or on mollusks such as clams. Most sea urchins are herbivores and gaze on algae. Brittle stars, sea lilies and sea cucumbers feeds on dead and decaying matter that drifts down to the ocean floor.
7
reproduction
• _______ reproduction • A Echinoderm is a male or female. The males and females discharge their eggs and sperm into the water where they are fertilized. • ______ reproduction • Many echinoderms have remarkable powers of regeneration. If a piece of certain echinoderms is chopped off, a new piece or even a new echinoderm can regrow.
3
Echinoderm
• _______________larvae at larval stages • Adult _____________enables animals to sense potential food, predators and other aspects of their environment from direction.
EFDC翻译
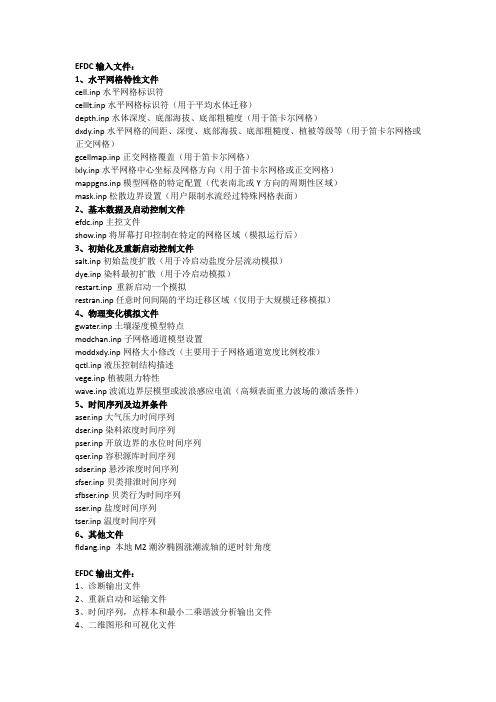
qctl.inp液压控制结构描述
vege.inp植被阻力特性
wave.inp波流边界层模型或波浪感应电流(高频表面重力波场的激活条件)
5、时间序列及边界条件
aser.inp大气压力时间序列
dser.inp染料浓度时间序列
pser.inp开放边界的水位时间序列
注:经纬度是地理坐标系统中定义地球上的坐标点,它是一个球面的坐标体系。而世界坐标系是一个以地心为圆心的正交直角坐标系。通常的数学算法,都是在直角坐标系下进行,因此在水环境模拟的时候,需要在地理坐标系下指定计算原点,并将该点的经纬度信息转换为直角坐标系下的数值,在完成计算后,将水体的移动位置的直角坐标数值转换为地理坐标系下的经纬度数值。
旋转矩阵的逆矩阵
lxly.inp
I表示行索引;J表示列索引;XLNUTME表示X轴的中心坐标(经度/米(千米));YLTUTMN表示Y轴的中心坐标(经度/米(千米));CCUE、CCVE、CCUN、CCVN表示旋转矩阵参数。
在实际的应用中,模型程序会生成一些预处理程序gefdc.f,用于生成水平网格,并形成dxdy.inp和lxly.inp文件。gefdc.f代码需要以下输入文件支持:
cell.inp网格文件
depdat.inp定义深度或者地形
gcell.inp网格文件的辅助文件,当实际计算的网格是曲线时,该文件会为矩阵定义一个辅助的卡迪尔网格
gridext.inp使用NTYPE = 0网格时,水域网格的角坐标文件生成选项
gefdc.inp主配置文件
vege.inp植被等级
zroቤተ መጻሕፍቲ ባይዱgh.inp底部粗糙度等级
lxly.inp水平网格中心坐标及网格方向(用于笛卡尔网格或正交网格)
岩心荧光 含油级别
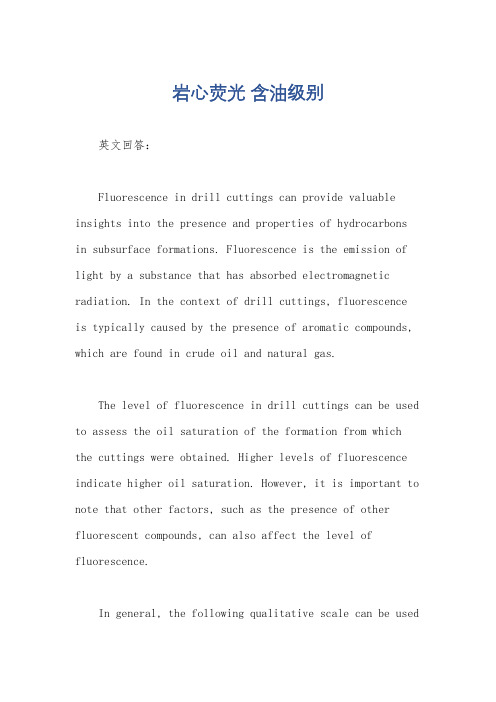
岩心荧光含油级别英文回答:Fluorescence in drill cuttings can provide valuable insights into the presence and properties of hydrocarbonsin subsurface formations. Fluorescence is the emission of light by a substance that has absorbed electromagnetic radiation. In the context of drill cuttings, fluorescence is typically caused by the presence of aromatic compounds, which are found in crude oil and natural gas.The level of fluorescence in drill cuttings can be used to assess the oil saturation of the formation from which the cuttings were obtained. Higher levels of fluorescence indicate higher oil saturation. However, it is important to note that other factors, such as the presence of other fluorescent compounds, can also affect the level of fluorescence.In general, the following qualitative scale can be usedto assess the oil saturation of drill cuttings based on their fluorescence:Non-fluorescent: No oil saturation.Weakly fluorescent: Low oil saturation.Moderately fluorescent: Moderate oil saturation.Strongly fluorescent: High oil saturation.It is important to note that this scale is only a general guideline and that the actual oil saturation of a formation may vary depending on a number of factors, such as the type of oil and the formation properties.In addition to assessing oil saturation, fluorescencein drill cuttings can also be used to identify the type of oil present. Different types of oil have different fluorescence characteristics, which can be used to differentiate between them. For example, crude oiltypically has a yellow-green fluorescence, while condensatehas a blue-white fluorescence.Fluorescence in drill cuttings is a valuable tool for formation evaluation. It can be used to assess the oil saturation of a formation, identify the type of oil present, and provide insights into the formation's properties.中文回答:岩心荧光是指岩心在受到光波激发后产生的光。
Ocean Optics 光谱仪 FPG 固件版本 3.0 及以上的新外部触发选项说明书
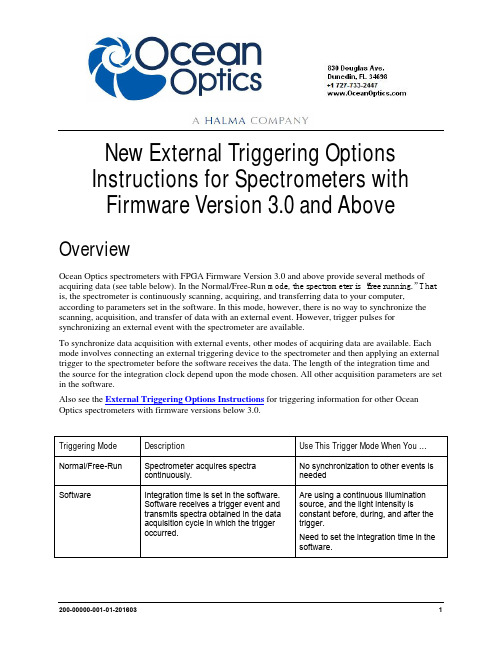
New External Triggering OptionsInstructions for Spectrometers withFirmware Version 3.0 and Above OverviewOcean Optics spectrometers with FPGA Firmware Version 3.0 and above provide several methods of acquiring data (see table below). In the Normal/Free-Run mode, the spectrometer is “free running.” That is, the spectrometer is continuously scanning, acquiring, and transferring data to your computer, according to parameters set in the software. In this mode, however, there is no way to synchronize the scanning, acquisition, and transfer of data with an external event. However, trigger pulses for synchronizing an external event with the spectrometer are available.To synchronize data acquisition with external events, other modes of acquiring data are available. Each mode involves connecting an external triggering device to the spectrometer and then applying an external trigger to the spectrometer before the software receives the data. The length of the integration time and the source for the integration clock depend upon the mode chosen. All other acquisition parameters are set in the software.Also see the External Triggering Options Instructions for triggering information for other Ocean Optics spectrometers with firmware versions below 3.0.Triggering Mode Description Use This Trigger Mode When You …Normal/Free-Run Spectrometer acquires spectracontinuously.No synchronization to other events is neededSoftware Integration time is set in the software.Software receives a trigger event andtransmits spectra obtained in the dataacquisition cycle in which the triggeroccurred.Are using a continuous illumination source, and the light intensity is constant before, during, and after the trigger.Need to set the integration time in the software.External Triggering Options Instructions for FW3.0and AboveTriggering Mode Description Use This Trigger Mode When You …External Hardware Level Trigger Integration time set via software on achip in spectrometer. The spectrometerwaits for a sharp rise in voltage on thetrigger input pin, and then acquiresspectra until the voltage is removed.Need a continuous acquisitionwhenever a certain condition is met,such as:-when reacting to a sample beingpresent or-when a sample reaches a specificstate that you want to measureExternal Synchronous Trigger Spectrometer acquires data from anexternal trigger event (such as a pushbutton) until the next time the trigger isactivated, at which time thespectrometer ceases spectralacquisition and begins a newacquisition. Integration time cannot beset, since the trigger can fire at randomintervals.Must synchronize your scans to anexternal clock sourceAre using a lock-in amplifierAre using a chopperExternal Hardware Edge Trigger Integration time set via software on achip in spectrometer. The spectrometerwaits for a sharp rise in voltage on thetrigger input pin, and then acquiresspectra.This trigger acquires onespectrum each time that there is asharp rising edge (if an acquisition isnot already in progress).Are using a pulsed excitation source orlight source in your experiment (suchas a laser or flash lamp)Are doing LIF (fluorescence withpulsed excitation) or phosphorescenceexperimentsNeed to synchronize an acquisitionwith an external eventSetting Up for External Triggering IMPORTANT:Voltage RegulationThe maximum recommended voltage on the Triggering Pin is 5.5 V.If your triggering device exceeds this voltage, you must regulate or condition the signal (via transistor buffering, transformer isolation or opto-isolation, for example) or isolate the signal from the spectrometer.NoteTo use one of the External Triggering options, you must know the specifications andlimitations of the triggering device. The design of the triggering device may prevent youfrom using one of the external triggering modes as it is described in these pages.External Triggering Instructions for FW3.0and Above Pinout Diagrams for Ocean Optics SpectrometersHR4000,HR2000+,Maya2000Pro and Maya-LSL and Maya-LSL,NIRQuest,QE65000, QE65Pro, and QE ProFor external triggering,supply a line from Pin 10 of the multi-pin connector on the spectrometer to your triggering device.Be sure to also use the Ground Pin when triggering. See the appropriate spectrometer Data Sheet to locate the ground pin(s).Pin-out Diagram of 30-pin Connector on HR4000,HR2000+,NIRQuest,Maya2000Pro and Maya-LSL,QE65000, QE65Pro,and QE Pro SpectrometersUSB2000+ and USB4000For external triggering, supply a line from Pin 7 of the multi-pin connector on the spectrometer to your triggering device. Be sure to also use Ground Pin 6 when triggering.Pin-out Diagram of 22-pin Connector on USB2000+, Flame-S,USB4000, and Flame-T SpectrometersFlame-S,Flame-T, and Flame-NIRFor external triggering, supply a line from Pin 2of the multi-pin connector on the spectrometer to your triggering device. Be sure to also use Ground Pin 1when triggering.When facing the 40-pin Accessory Connector on the front of the vertical wall of the Flame, pinnumber 1 is on the right.Pin-out Diagram of40-pin Connector on Flame-S,Flame-T and Flame-NIR SpectrometersExternal Triggering Options Instructions for FW3.0and AboveSetting Integration Time in SoftwareSoftware,External Hardware Level Trigger and External Hardware Edge Trigger modes can have the integration time set via OceanView or SpectraSuite.Set Integration Time with OceanViewUse the Main Controls of the Set Acquisition Parameters in OceanView to select the trigger mode and to set the integration time.The Automatic button in the Integration Time control box automatically adjusts the spectrometer’s integration time to bring the highest point of the signal to 85% of full saturation value. This value is represented by the horizontal blue line on the preview graph.External Triggering Instructions for FW3.0and AboveThe integration time may also be set manually by using the numeric spinner control and the drop-down units menu.Note that the Nonlinearity Correction control is grayed out until the Electric Dark control is enabled.Also note that once a triggering mode for the spectrometer has been set, the device will wait for an external trigger before continuing. It will appear as though the spectrometer has locked up and no further data acquisitions will occur until the External Trigger pin on the device receives a signal.External Triggering Options Instructions for FW3.0and AboveSet Integration Time with SpectraSuiteUse the External Trigger selection box on the Trigger toolbar to set the trigger mode of the spectrometer to Software.NoteOnce you select an external trigger mode, your computer will appear unresponsive. Thisis normal, as the computer is waiting for a trigger. You must apply one more trigger tothe spectrometer after selecting a new trigger mode.External Triggering vs. Triggering an External EventThere could be some confusion between the concepts of External Triggering and triggering an external event. The following sections explain each of these concepts:∙External Triggering–An event outside the sampling system (such as a push button, lever activation, or laser pulse) triggers the voltage level on the spectrometer’s trigger pin and instructs the spectrometer to begin spectra acquisition.∙Triggering an External Event–When triggering an external event, the spectrometer instructs an external device (typically a lamp such as the PX-2 or the LS-450) to illuminate immediately prior to spectral acquisition.Trigger Mode DescriptionsThe following sections specify the Trigger modes for Ocean Optics spectrometers with firmware versions 3.0 and above and associated timing sequences.For the Maya2000Pro and Maya-LSL,NIRQuest,Flame-NIR,HR2000+,USB2000+, Flame-S,HR4000, USB4000,and Flame-T,the timing sequences specified are for the trigger mechanism interacting with a single-depth FIFO.The hardware implementing these Trigger modes may enhance the capability and performance by implementing buffering schemes using larger or multiple FIFOs.For the QE65000,the timing sequences specified are for the trigger mechanism interacting with a triple-depth FIFO. The hardware implementing these Trigger modes enhances the capability and performance by implementing a buffering scheme using multiple FIFOs.In addition, the QE Pro has a 15,698-deep buffer.External Triggering Instructions for FW3.0and Above Normal ModeIn the Normal (Free-run) mode, the spectrometer will acquire one spectrum for each “get spectrum”command issued from the host software.The integration time for each spectrum is pre-programmed prior to the host issuing its “get spectrum” command.The spectrometer waits in a repetitive idling loop until it receives the next “get spectrum” command.Once the command is received, the spectrometer exposes the detector for the pre-programmed integration time, and its resulting spectrum is written to an internal FIFO.The spectrometer signals the host that a spectrum is ready, and the host reads out the new spectrum.Upon reading out the new spectrum, the host immediately commands the spectrometer to acquire the next spectrum.In this process, the spectrometer is responding “on-demand”to the host’s “get spectrum” commands.The spectrometer waits in an idle state between each spectrum and the next “get spectrum” command.The spectrometer does not pre-fetch any spectra in anticipation of the next “get spectrum” command.For the QE65000spectrometer, integrations are continuously performed with the most recent three spectra available to the host software. The QE65000 has an internal FIFO which can hold up to three contiguous spectra.To maximize throughput, the QE6500 is constantly pre-fetching spectra back-to-back, independent of the host’s “get spectrum” command.However, if the host fails to issue a “get spectrum” command by the time the third spectrum is pre-fetched, the QE65000 clears its FIFO and starts 3 new acquisitions.In this process, the QE65000 is pre-fetching back-to-back spectra in anticipation of the host’s “get spectrum” command.For the QE Pro, there are no idle cycles that drop new spectra; all new spectra are stored in the buffer and are available to the user (if buffering is enabled). If the buffer limit is exceeded (15,698), the oldest spectrum is automatically discarded to make room for the new spectrum. The user can clear the buffer at any time.External Triggering Options Instructions for FW3.0and AboveNormal Mode Timing SequenceSoftware Trigger ModeIn this level-triggered mode, the spectrometer is “free running,” just as it is in the Normal mode. The spectrometer is continually scanning and collecting data. With each trigger, the data collected up to the trigger event is transferred to the software. If you continuously apply triggers (for example, by holding down the button on via an external switch), this mode is equivalent to operating in the Normal mode.In the Software Trigger mode, you set the integration time (as well as all other acquisition parameters) in the software.The source for the integration clock comes from the A/D converter.External Triggering Instructions for FW3.0and Above If the software trigger is asserted during integration cycle n, the photons from this integration period will be readout and digitized at the start of integration cycle n+1External Software Triggering–Trigger TimingExternal Synchronous Trigger ModeIn the External Synchronous Trigger Mode, two external triggers are required to complete a data acquisition. The first rising edge starts the integration period and the second rising edge stops the integration while starting the next integration. Thus the integration time is the period between the two external trigger pulses. After the each integration period, the spectra is retrieved and written to the FIFO in the FPGA.For the Maya2000Pro and Maya-LSL, HR2000+,USB2000+,Flame-S and Flame-NIR,as in all nonbuffered modes, no further integrations are possible until the software has read the entire contents of the FIFO.For the QE65000, three spectrum buffers provide software with the most recent spectral acquisitions.External Triggering Options Instructions for FW3.0and AboveExternal Synchronous Trigger Mode Timing SequenceExternal Hardware Level Trigger ModeIn the External Hardware Level Trigger mode, a rising edge detected by the FPGA from the External Trigger input starts the Integration Cycle specified through the software interface. After the Integration Cycle completes, the spectrum is retrieved and written to the FIFO in the FPGA. As long as the trigger level remains active in a logic one state, continuous acquisitions will occur with the following exception. Each subsequent acquisition must wait until a minimum CCD Reset Cycle completes. This Reset Cycle insures that the CCD performance uniform on a scan-to-scan basis. The time duration for this reset cycle is relative to the Integration Cycle time and will change if the integration period is changed. So the timing sequence is Trigger, Trigger Delay,Integration Cycle, Read/Write Cycle, Reset Cycle, Idle Cycle(s), and Integration Cycle (if trigger is still high). The Idle Cycle will on last 2µs if the trigger remains high and the FIFO is empty and a spectrum request is active, otherwise the Idle Cycle will continue until all 3 conditions are satisfied.For the Maya2000Pro and Maya-LSL, HR2000+,USB2000+,Flame-S and Flame-NIR, as in all nonbuffered modes, no integrations are possible until the software has read the entire contents of the FIFO.External Hardware Level Trigger Mode Timing SequenceFor the QE65000, three spectrum buffers provide software with the most recent spectral acquisitions.QE65000 Hardware Level Trigger ModeQE Pro Hardware Level Trigger ModeExternal Hardware Edge Trigger ModeIn the External Hardware Edge Trigger mode, a rising edge detected by the FPGA from the External Trigger input starts the Integration Cycle specified through the software interface. After the Integration Cycle completes, the spectrum is retrieved and written to the FIFO in the FPGA followed by a CCD Reset Cycle. Only one acquisition will be performed for each External Trigger pulse, no matter what thepulse’s duration is. The Reset Cycle insures that the CCD performance uniform on a scan-to-scan basis. The time duration for this reset cycle is relative to the Integration Cycle time and will change if the integration period is changed. So the timing sequence is Trigger, Trigger Delay, Integration Cycle, Read/Write Cycle, Reset Cycle, and Idle Cycle(s). The Idle Cycle will until the next trigger occurs.NoteFor the QE Pro, jitter between external edge trigger and start of column binning (ortrigger delay) is 40ns.Maya2000Pro and Maya-LSL,and QE65000 Hardware Edge Trigger Mode Time TableQE Pro Hardware Edge Trigger Mode Time TableHR2000+,USB2000+and Flame-S Hardware Edge Trigger Mode Time TableNIRQuest Hardware Trigger Mode Time TableHR4000,USB4000and Flame-T TimetablesIt is important to note that the trigger timing in the USB4000and Flame-T will vary depending upon the integration time.This is further detailed in the Time Tables that appear below. These tables reveal that the trigger timing will be different for the following ranges of integration times:∙< 3.8 ms∙ 3.8 ms to 199 ms∙200 ms to 2097 ms∙2097 msUSB Command Description for TriggeringThe USB command Set Trigger Mode sets the spectrometer trigger to one of the trigger mode states as shown below.HR2000+,USB2000+and Flame-S Set Trigger ModeData Value = 0→Normal (Free running) ModeData Value = 1→Software Trigger ModeData Value = 2→External Hardware Level Trigger ModeData Value = 3→External Synchronization Trigger ModeData Value = 4→External Hardware Edge Trigger ModeHR4000,USB4000and Flame-T Set Trigger ModeData Value = 0→Normal (Free running) ModeData Value = 1→Software Trigger ModeData Value = 2→External Hardware Level Trigger ModeData Value = 3→Normal(Shutter)ModeData Value = 4→External Hardware Edge Trigger ModeMaya2000Pro and Maya-LSL,QE65000, QE65 Pro, and QE Pro Set Trigger ModeData Value = 0→Normal (Free running) ModeData Value = 1→External Hardware Level Trigger ModeData Value = 2→External Synchronous Trigger Mode*Data Value = 3→External Hardware Edge Trigger Mode*Not yet implemented on the QE ProNIRQuest Set Trigger ModeData Value = 0→Normal (Free running) ModeData Value = 3→External Hardware Edge Trigger Mode。
低频活动漂浮潜水船声探测系统(LFATS)说明书

LOW-FREQUENCY ACTIVE TOWED SONAR (LFATS)LFATS is a full-feature, long-range,low-frequency variable depth sonarDeveloped for active sonar operation against modern dieselelectric submarines, LFATS has demonstrated consistent detection performance in shallow and deep water. LFATS also provides a passive mode and includes a full set of passive tools and features.COMPACT SIZELFATS is a small, lightweight, air-transportable, ruggedized system designed specifically for easy installation on small vessels. CONFIGURABLELFATS can operate in a stand-alone configuration or be easily integrated into the ship’s combat system.TACTICAL BISTATIC AND MULTISTATIC CAPABILITYA robust infrastructure permits interoperability with the HELRAS helicopter dipping sonar and all key sonobuoys.HIGHLY MANEUVERABLEOwn-ship noise reduction processing algorithms, coupled with compact twin line receivers, enable short-scope towing for efficient maneuvering, fast deployment and unencumbered operation in shallow water.COMPACT WINCH AND HANDLING SYSTEMAn ultrastable structure assures safe, reliable operation in heavy seas and permits manual or console-controlled deployment, retrieval and depth-keeping. FULL 360° COVERAGEA dual parallel array configuration and advanced signal processing achieve instantaneous, unambiguous left/right target discrimination.SPACE-SAVING TRANSMITTERTOW-BODY CONFIGURATIONInnovative technology achievesomnidirectional, large aperture acousticperformance in a compact, sleek tow-body assembly.REVERBERATION SUPRESSIONThe unique transmitter design enablesforward, aft, port and starboarddirectional transmission. This capabilitydiverts energy concentration away fromshorelines and landmasses, minimizingreverb and optimizing target detection.SONAR PERFORMANCE PREDICTIONA key ingredient to mission planning,LFATS computes and displays systemdetection capability based on modeled ormeasured environmental data.Key Features>Wide-area search>Target detection, localization andclassification>T racking and attack>Embedded trainingSonar Processing>Active processing: State-of-the-art signal processing offers acomprehensive range of single- andmulti-pulse, FM and CW processingfor detection and tracking. Targetdetection, localization andclassification>P assive processing: LFATS featuresfull 100-to-2,000 Hz continuouswideband coverage. Broadband,DEMON and narrowband analyzers,torpedo alert and extendedtracking functions constitute asuite of passive tools to track andanalyze targets.>Playback mode: Playback isseamlessly integrated intopassive and active operation,enabling postanalysis of pre-recorded mission data and is a keycomponent to operator training.>Built-in test: Power-up, continuousbackground and operator-initiatedtest modes combine to boostsystem availability and accelerateoperational readiness.UNIQUE EXTENSION/RETRACTIONMECHANISM TRANSFORMS COMPACTTOW-BODY CONFIGURATION TO ALARGE-APERTURE MULTIDIRECTIONALTRANSMITTERDISPLAYS AND OPERATOR INTERFACES>State-of-the-art workstation-based operator machineinterface: Trackball, point-and-click control, pull-down menu function and parameter selection allows easy access to key information. >Displays: A strategic balance of multifunction displays,built on a modern OpenGL framework, offer flexible search, classification and geographic formats. Ground-stabilized, high-resolution color monitors capture details in the real-time processed sonar data. > B uilt-in operator aids: To simplify operation, LFATS provides recommended mode/parameter settings, automated range-of-day estimation and data history recall. >COTS hardware: LFATS incorporates a modular, expandable open architecture to accommodate future technology.L3Harrissellsht_LFATS© 2022 L3Harris Technologies, Inc. | 09/2022NON-EXPORT CONTROLLED - These item(s)/data have been reviewed in accordance with the InternationalTraffic in Arms Regulations (ITAR), 22 CFR part 120.33, and the Export Administration Regulations (EAR), 15 CFR 734(3)(b)(3), and may be released without export restrictions.L3Harris Technologies is an agile global aerospace and defense technology innovator, delivering end-to-endsolutions that meet customers’ mission-critical needs. The company provides advanced defense and commercial technologies across air, land, sea, space and cyber domains.t 818 367 0111 | f 818 364 2491 *******************WINCH AND HANDLINGSYSTEMSHIP ELECTRONICSTOWED SUBSYSTEMSONAR OPERATORCONSOLETRANSMIT POWERAMPLIFIER 1025 W. NASA Boulevard Melbourne, FL 32919SPECIFICATIONSOperating Modes Active, passive, test, playback, multi-staticSource Level 219 dB Omnidirectional, 222 dB Sector Steered Projector Elements 16 in 4 stavesTransmission Omnidirectional or by sector Operating Depth 15-to-300 m Survival Speed 30 knotsSize Winch & Handling Subsystem:180 in. x 138 in. x 84 in.(4.5 m x 3.5 m x 2.2 m)Sonar Operator Console:60 in. x 26 in. x 68 in.(1.52 m x 0.66 m x 1.73 m)Transmit Power Amplifier:42 in. x 28 in. x 68 in.(1.07 m x 0.71 m x 1.73 m)Weight Winch & Handling: 3,954 kg (8,717 lb.)Towed Subsystem: 678 kg (1,495 lb.)Ship Electronics: 928 kg (2,045 lb.)Platforms Frigates, corvettes, small patrol boats Receive ArrayConfiguration: Twin-lineNumber of channels: 48 per lineLength: 26.5 m (86.9 ft.)Array directivity: >18 dB @ 1,380 HzLFATS PROCESSINGActiveActive Band 1,200-to-1,00 HzProcessing CW, FM, wavetrain, multi-pulse matched filtering Pulse Lengths Range-dependent, .039 to 10 sec. max.FM Bandwidth 50, 100 and 300 HzTracking 20 auto and operator-initiated Displays PPI, bearing range, Doppler range, FM A-scan, geographic overlayRange Scale5, 10, 20, 40, and 80 kyd PassivePassive Band Continuous 100-to-2,000 HzProcessing Broadband, narrowband, ALI, DEMON and tracking Displays BTR, BFI, NALI, DEMON and LOFAR Tracking 20 auto and operator-initiatedCommonOwn-ship noise reduction, doppler nullification, directional audio。
Cai_2001_Comparison-of--solid-pmicroextraction-for-the-determination-of-volatile-flavor-components
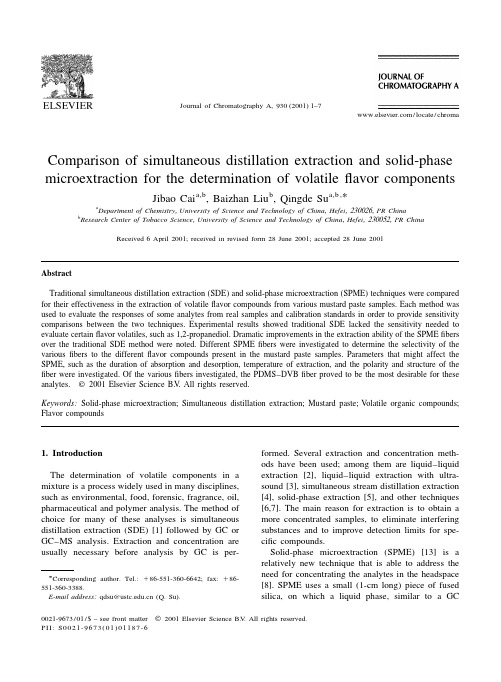
930 (2001) 1–7Journal of Chromatography A,/locate/chromaComparison of simultaneous distillation extraction and solid-phase microextraction for the determination of volatile flavor componentsa,b b a,b ,*Jibao Cai ,Baizhan Liu ,Qingde SuaDepartment of Chemistry ,University of Science and Technology of China ,Hefei ,230026,PR ChinabResearch Center of Tobacco Science ,University of Science and Technology of China ,Hefei ,230052,PR ChinaReceived 6April 2001;received in revised form 28June 2001;accepted 28June 2001AbstractTraditional simultaneous distillation extraction (SDE)and solid-phase microextraction (SPME)techniques were compared for their effectiveness in the extraction of volatile flavor compounds from various mustard paste samples.Each method was used to evaluate the responses of some analytes from real samples and calibration standards in order to provide sensitivity comparisons between the two techniques.Experimental results showed traditional SDE lacked the sensitivity needed to evaluate certain flavor volatiles,such as 1,2-propanediol.Dramatic improvements in the extraction ability of the SPME fibers over the traditional SDE method were noted.Different SPME fibers were investigated to determine the selectivity of the various fibers to the different flavor compounds present in the mustard paste samples.Parameters that might affect the SPME,such as the duration of absorption and desorption,temperature of extraction,and the polarity and structure of the fiber were investigated.Of the various fibers investigated,the PDMS–DVB fiber proved to be the most desirable for these analytes.©2001Elsevier Science B.V .All rights reserved.Keywords :Solid-phase microextraction;Simultaneous distillation extraction;Mustard paste;Volatile organic compounds;Flavor compounds1.Introductionformed.Several extraction and concentration meth-ods have been used;among them are liquid–liquid The determination of volatile components in a extraction [2],liquid–liquid extraction with ultra-mixture is a process widely used in many disciplines,sound [3],simultaneous stream distillation extraction such as environmental,food,forensic,fragrance,oil,[4],solid-phase extraction [5],and other techniques pharmaceutical and polymer analysis.The method of [6,7].The main reason for extraction is to obtain a choice for many of these analyses is simultaneous more concentrated samples,to eliminate interfering distillation extraction (SDE)[1]followed by GC or substances and to improve detection limits for spe-GC–MS analysis.Extraction and concentration are cific compounds.usually necessary before analysis by GC is per-Solid-phase microextraction (SPME)[13]is a relatively new technique that is able to address the need for concentrating the analytes in the headspace *Corresponding author.Tel.:186-551-360-6642;fax:186-[8].SPME uses a small (1-cm long)piece of fused 551-360-3388.E -mail address :qdsu@ (Q.Su).silica,on which a liquid phase,similar to a GC0021-9673/01/$–see front matter ©2001Elsevier Science B.V .All rights reserved.PII:S0021-9673(01)01187-6930 (2001) 1–72J.Cai et al./J.Chromatogr.Astationary phase,has been coated to absorb the waterbath maintained at30,50and708C,respective-desired analytes and concentrate them on thefiber.ly,to optimize temperature of extraction.The vial The selectivity of the extraction of target analytes in was submerged only as far as necessary to submerge the gaseous phase can be significantly altered the solid phase of the sample,to help keep the SPME through the use of different liquid phase on thefiberfiber cool,which is a desired condition for SPME.[9].This is because as the temperature of thefiber Mustard paste,which is usually served as a spice increases,the partition coefficient decreases[10]. for foodflavoring,has become increasingly popular.The SPME holder was secured and thefiber extend-The main ingredients of mustard paste are Brassica ed into the headspace,and thefiber was equilibrated nigra and Brassica alba seeds.The most predomi-for20,40and60min,respectively,to optimize the nant constituent of Brassica nigra is allyl isothio-time of extraction.Thefiber was then retracted, cyanate,which accounts for more than90%of the removed from the vial,and placed immediately into total volatile compounds.The most predominant the injector of the GC at2508C.Injection was constituent of Brassica alba is sinalbin disulfide.accomplished by extending thefiber in the heated Consequently,the analysis of the volatileflavor inlet for3,5and7min,to optimize the time of compounds in mustard paste can identify the mustard desorption,while the injector operated in the splitless varieties.mode for2min.The additional time of exposuretime in the injector port allowed thefiber to becleaned of any compounds that may not be desorbed 2.Materials and methods in the initial minute.Preliminary studies indicatedthat the above procedure allowed for reproducible, 2.1.Materials quantitative transfer of target analytes into the injec-tor port of the GC–MS.Mustard pastes(made in Japan)were purchasedfrom a local supermarket.The mustard paste is2.3.Sampling conditions of SDEcomposed of mustard,sorbitol,corn oil,salt,water,artificialflavor,xanthan gum,turmeric,and artificialSimultaneous distillation–solvent extraction was color(FD&C Yellow no.5,FD&C Blue no.1).Thecarried out in a microversion apparatus,as described components of the standard solutions were all pur-elsewhere[11].Dichloromethane(chromatography-chased from Sigma(St.Louis,MO,USA).Standardgrade reagent,Merck)and n-tetradecane were used solutions were used to optimize GC–MS and SPMEas solvent and internal standard,respectively.For conditions.All solutions were stored at48C.each extraction,10g of mustard paste and250mldistilled water were placed in a500-mlflask,30ml 2.2.Sampling conditions of SPMEdichloromethane was placed in a50-mlflask,streamdistillation was stopped after2h,while the solvent For the SPME determinations,a SPME holder andextraction was continued for a further15min.The threefibers(100-m m PDMS,65-m m PDMS–DVBextract was concentrated to 1.0ml at558C by and65-m m CW–DVB)were used(Supelco,Belle-Kuderna-Danish apparatus(NE-1,Japan).The in-fonte,PA,USA).Thefibers were conditioned underjection volume was2.0m l with a split ratio of20:1. helium at2908C for4–5h prior to use.BetweenA series of three consecutive extractions was per-uses,fibers were kept sealed from ambient air byformed on different aliquots of mustard pastes in inserting the tip of the SPME needle into a smallorder to evaluate the repeatability of SDE method. piece of septum to prevent accidental contamination.The sampling procedure involved placing2–3g ofsample into a20-ml vial and sealing with a screwtop 2.4.Condition of GC–MSseptum-containing cap.The SPME needle was theninserted through the septum and suspended in the Autosystem TurboMass GC–MS(Perkin-Elmer, headspace of the vial.The vial was placed in a USA)was used.A30m30.25mm Supelco-wax930 (2001) 1–73J.Cai et al./J.Chromatogr.Aquartz capillary column(Supelco,Bellefonte,PA, 3.Results and discussionUSA)with0.25-m mfilm thickness was used toresolve the volatiles with the following temperature parison of SDE and headspace SPME programming:initial oven temperature was608C,techniquekept for2min;then was raised to2408C at48C/min,and kept at2408C for15min.Helium was used as As shown in Table1,traditional SDE technique carrier gas with column head pressure at10kPa.could extract all the volatileflavor compounds of Programming split/splitless(PSS)injector tempera-mustard paste,except for1,pared ture was at2508C.In SPME analysis,the I.D.of the with SPME,SDE could also extract high-molecular-injection liner was1.5mm;the desorption time was mass and low volatility compounds such as oleic 5min in splitless mode;and the time of the splitless acid,9-hexadecenoic acid and palmitic acid in was2min.In the analysis of SDE extract,the I.D.of volatileflavor compounds of mustard paste.So the the injection liner was4.0mm;the split ratio was traditional SDE technique seemed more comprehen-20:1,and the amount of injection was2m l.The sive but less sensitive to trace rge temperature of the GC–MS transfer line was2508C.amounts of furfural and furfural alcohol were found The MS was operated at1708C in the electron in the SDE extracts,perhaps arising from pyrolysis impact mode(70eV),scanning from m/z33to350or hydrolysis during the SDE process.In fact,if in0.3s with an0.2-s interval time of the scan;the these compounds were mustard paste volatileflavor voltage of the photoelectric multiplier tube(PMT)components,they should easily be extracted by was230V.The mass spectral identifications of the SPME.However,they were found only in SDE volatiles were carried out by comparing to the extracts.Representative TIC chromatograms of vola-NIST98(National Institute of Standards and Tech-tileflavor compounds from mustard pastes are shown nology,Gaithersburg,MD,USA)mass spectral in Fig. pared with SDE,SPME showed library as well as to the Wiley6.0(Wiley,New York,enormous advantages:simplicity,rapid solvent-free NY,USA)mass spectral library.extraction,low cost,little interference,no apparentTable1GC–MS identification of mustard paste volatiles and peak area percentagesPeak t Compound name Peak area(%)Rno.(min)100-m m65-m m65-m m SDEPDMS CW–DVB PDMS–DVB1 5.081-Propene,3,3-thiobis0.0220.0130.0170.009 28.10Thiocyanic acid,methyl ester0.0160.0270.0210.011 313.56Allyl isothiocyanate98.5863.6293.2498.84 413.83Furfural ND ND ND0.081 514.20Diallyl disulfide0.0220.0080.0130.012 617.50Methyl allyl trisulfide0.0140.0120.0130.006 717.881,2-Propanediol 1.12835.77 3.125ND 819.87Furfural alcohol ND ND ND0.125 923.37Diallyl trisulfide0.0150.0140.0140.005 1026.93Butylated hydroxyl toluene0.0700.0130.0410.011 1128.475-Methyl-tetrahydrothiophen-2-one0.0510.3300.1950.014 1231.25Ethanol,1-methoxy-,benzoate0.0090.0410.0270.011 1334.722-Phenylethyl isothiocyanate0.0140.0210.0160.012 1449.67Palmitic acid ND ND ND0.508 1550.619-Hexadecenoic acid ND ND ND0.799 1650.90Oleic acid ND ND ND 1.522 ND,not determined.930 (2001) 1–7 4930 (2001) 1–75J.Cai et al./J.Chromatogr.ATable2Repeatability of SPME(n55)and SDE(n53)Peak Compound name RSD(%)no.100m m65m m65m m SDECW–DVB PDMS PDMS–DVB11-Propene,3,3-thiobis 6.23 3.71 6.74 2.032Thiocyanic acid,methyl ester 5.768.73 4.73 3.743Allyl isothiocyanate8.64 3.52 2..22 1.614Furfural––– 3.495Diallyl disulfide 5.66 5.758.08 2.536Methyl allyl trisulfide–8.779.24 3.6871,2-Propanediol 4.60 4.207.99–8Furfural alcohol––– 1.369Allyl trisulfide7.919.72 3.29 2.2710Butylated hydroxyl toluene 5.817.55 5.82 3.52115-Methyl-tetrahydrothiophen-2-one9.557.44 4.29 2.4512Ethanol,1-methoxy-,benzoate7.91 4.75 6.48 1.45132-Phenylethyl isothiocyanate 6.689.148.89 2.3714Palmitic acid––– 3.09159-Hexadecenoic acid––– 1.3416Oleic acid––– 2.15–,not determined.21less than0.3m g l for most of analytes.The aqueous layer at thefiber-gas interface increased relative standard deviation(RSD)is better than9%.with increasing temperature,so that more analytes This method was applied to a food sample(mustard were absorbed at higher temperature if equilibrium paste)using an external calibration.had not been reached.The decreasing absorptionwith increasing temperature at708C was presumably 3.2.1.Extraction temperature due to the distribution constant decreasing with The extraction temperature profile obtained using increasing temperature.The absorption process was a PDMS–DVBfiber is shown in Fig.2.Optimum exothermic,thus lowing the temperature increased extraction efficiency was achieved at508C.The the distribution constant at equilibrium.In practical lower absorption of most analytes at308C was due to applications when the extraction was stopped before the decreased rate of diffusion of the analytes.The reaching the equilibrium,not only thermodynamic rate of diffusion of the analytes through the static but also kinetic aspects became important.An ex-traction temperature of508C was selected for thisstudy using the threefibers,because this temperaturewas relatively easily maintained,and the improve-ment in sensitivity at higher temperature was notnecessary.3.2.2.Extraction timeThe extraction time profile obtained using PDMS–DVBfiber is shown in Fig.3.For the PDMS–DVBfiber,the equilibrium condition for the absorption ofthe most analytes was almost reached after40min.Factors that influenced the equilibration period wereinvestigated by Pawliszyn and co-workers[13–15].The equilibration rate was limited by(1)the masstransfer rate of the analytes through a thin static Fig.2.Extraction temperature profile for65-m m PDMS–DVBfiber.Extraction time,40min;desorption time,5min.aqueous layer at thefiber-gas interface,(2)the930 (2001) 1–76J.Cai et al./J.Chromatogr.A3.2.parison of differentfibersThree differentfibers were evaluated to determinewhichfiber most effectively extractedflavor volatilesfrom mustard paste samples.Thefibers that wereused to extract analytes from the headspace ofaliquots of the same sample for comparison of therelative extraction effectiveness were100-m mPDMS,65-m m CW–DVB and65-m m PDMS–DVB,respectively.The results of the experiments on thesethreefibers are summarized in Fig.5.These resultsshow that,of thefibers evaluated,the PDMS–DVBfiber proved to be the most effective in extractingflavor volatiles overall,followed by the PDMSfiber,then the CW–DVBfiber.Therefore,the65-m m Fig. 3.Extraction time profile for65-m m PDMS–DVBfiber.Extraction temperature,508C;desorption time,5min.PDMS–DVBfiber was used for all subsequentcomparison experiments.The PDMS–DVBfiber was distribution constant of thefiber coating and(3)the chosen as a representative to investigate the duration thickness and kinds of thefiber coating.Extraction of absorption and desorption,temperature of absorp-periods of40min were chosen for the threefibers tion,detection of limits,and the precision of SPME since it was approximately equivalent to the time in this investigation.required to run GC in this experiment.The PDMS–DVBfiber performed the most effec-tive extractions,for this analysis,because thefiber 3.2.3.Desorption time coating was composed of a mixed coating containing The desorption time profile obtained using the PDMS,a liquid phase that favored the absorption of PDMS–DVBfiber is shown in Fig.4.A desorption nonpolar analytes,as well as DVB,a porous solid period of5min was enough to desorb the analytes that favored the adsorption of the more polar ana-from the PDMS–DVBfiber(temperature of the GC lytes.There was little difference between the PDMS injection port,2508C).So a desorption period of5fiber and the PDMS–DVBfiber in extracting the min was used for the threefibers(temperature of the nonpolar analytes(butylated hydroxytoluene),but GC injection port,2508C).No carryover of any the more polar disulfide and trisulfide were extracted, volatileflavor component was observed.on average,three times better by the PDMS–DVBFig. 4.Desorption time profile for65-m m PDMS–DVBfiparison of100-m m PDMS,65-m m PDMS–DVB and Extraction temperature,508C;extraction time,40min.65-m m CW–DVBfibers.930 (2001) 1–77J.Cai et al./J.Chromatogr.Afiber as measured by peak area.The CW–DVBfiber,sitivity allowed fast,accurate determinations of which was more selective towards polar analytes,didflavor compounds and easy performance of analyses. show enhanced extraction effectiveness of the polar Consequently,SPME was suitable for simple,rapid, analytes,but was less effective with the nonpolar routine screening,while SDE was used for proper analytes.quantitative analysis.Profiling of different mustard paste samples were3.2.5.Repeatability performed.Differentfibers were investigated withA series offive consecutive extractions were the bestfiber found to be65-m m PDMS–DVB.The performed on different aliquots of mustard pastes in optimal parameters for SPME sampling were also order to evaluate the repeatability of the headspace investigated.SPME(HS-SPME)method.The precision of theHS-SPME method was good and the RSD valueswere between2.22and9.72%for all the11volatile Referencesflavor compounds in mustard paste(Table2).[1]A.Orav,T.Kailas,M.Liiv,Chromatography43(1996)215.3.3.Mustard pastes determined by SPME–GC–MS[2]D.Martinez,F.Borrul,M.Calull,J.Chromatogr.A827(1998)105.[3]T.Hankemeier,S.J.Kok,R.J.J.Vreuls,U.A.Th.Brinkman,J. In total,11volatile compounds in mustard pasteChromatogr.A841(1999)75.were identified,which accounts for99%of TIC peak[4]A.J.Nunez,J.M.H.Bemelman,J.Chromatogr.294(1984) area as shown in Table1.The four methods made up361.[5]T.Hankemeier,E.Hooijschuur,R.J.J.Vreuls,U.A.Th.Brink-one another and validated mutually.Since allylman,T.Visser,J.High Resolut.Chromatogr.21(1998)341. isothiocyanate was the main volatile constituents of[6]M.D.Burford,S.B.Hawthorne,ler,J.Chromatogr. the mustard pastes(Table1),the main ingredient ofA685(1994)79.the mustard pastes was Brassica nigra seeds.[7]B.Gawdzik,T.Matynia,Chromatography38(1994)643.[8]Z.Zhang,M.Yang,J.Pawliszyn,Anal.Chem.66(1994)844A.[9]X.Yang,T.Pepard,LC–GC13(1995)882.4.Conclusions[10]Z.Zhang,J.Pawliszyn,Anal.Chem.67(1995)34.[11]M.Godefroot,P.Sandra,M.Verzele,J.Chromatogr.203 Traditional SDE analysis of volatile compounds is(1981)325.a widely used technique.However,for many analy-[12]C.L.Arthur,L.Killam,K.Buchhliz,J.Pawliszyn,J.Berg,Anal.Chem.64(1992)1960.ses,the SDE method lacked the sensitivity and[13]C.L.Arthur,J.Pawliszyn,Anal.Chem.62(1990)2145. convenience needed to perform adequately.SPME[14]D.Louch,S.Motlagh,J.Pawliszyn,Anal.Chem.64(1992) had the ability to perform these analyses where SDE1187.fell parison of SDE and SPME showed[15]D.W.Potter,J.Pawliszyn,J.Chromatogr.625(1992)247. that SPME determinations offlavor compounds[16]D.C.Garcia,S.Magnaghi,M.Reinchenbacher,K.Danzer,J.High Resolut.Chromatogr.19(1996)257.were,on average,more sensitive under the con-ditions employed in this study.The increased sen-。
2SA733 半导体透封式PNP二极管技术参数与性能特性说明说明书
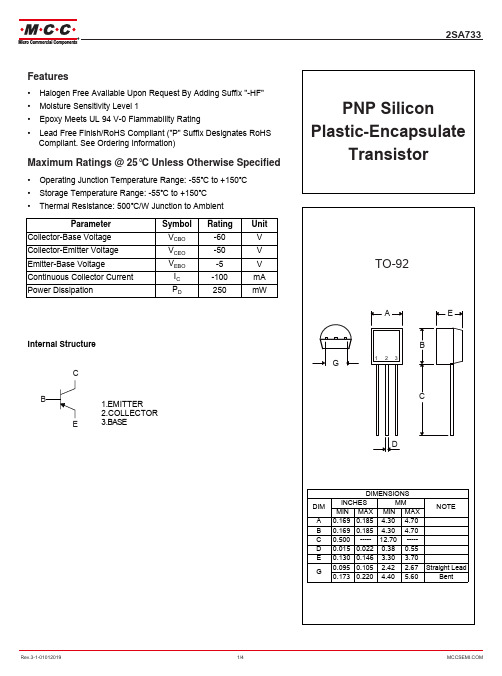
PNP Silicon Plastic-EncapsulateTransistorInternal StructureE1.EMITTER2.COLLECTOR3.BASE250mWFeatures•Halogen Free Available Upon Request By Adding Suffix "-HF"•Moisture Sensitivity Level 1•Epoxy Meets UL 94 V-0 Flammability Rating•Lead Free Finish/RoHS Compliant ("P" Suffix Designates RoHS Compliant. See Ordering Information)Collector-Base Voltage V CBO -60V •Operating Junction Temperature Range: -55℃ to +150℃•Storage Temperature Range: -55℃ to +150℃•Thermal Resistance: 500℃/W Junction to AmbientParameterSymbol Rating Unit Maximum Ratings @ 25°C Unless Otherwise SpecifiedCollector-Emitter Voltage V CEO -50V Emitter-Base Voltage V EBO -5V P DContinuous Collector Current I C -100mA Power DissipationElectrical Characteristics @ T A =25°C Unless Otherwise SpecifiedParameterSymbol Min TypMaxUnits ConditionsI C =-5µA, I E =0Collector-Emitter Breakdown Voltage V (BR)CEO -50V I C =-1mA, I B =0Collector-Base Breakdown Voltage V (BR)CBO -60V I E =-50µA, I C =0Collector-Base Cutoff Current I CBO -0.1µA V CB =-60V, I E =0Emitter-Base Breakdown Voltage V (BR)EBO -5V V EB =-5V, I C =0DC Current Gainh FE 90600V CE =-6V, I C =-1mA Emitter-Base Cutoff Current I EBO -0.1µAI C =-100mA, I B =-10mA Collector-Emitter Saturation Voltage V CE(sat)-0.18-0.30V V CE =-6V,I C =-0.3mA,f=100Hz,R g =10KΩClassification of h FENoise FigureN F20dBV CE =-6V,I C =-10mA Collector Output Capacitance C ob 6pF V CB =-10V,I E =0,f=1MHzTransition Frequency f T 100MHz 90-180135-270200-400300-600Rank R Q P K RangeCurve Characteristics-0-2-4-6-8-10-1-2-3-0-4C o l l e c t o r C u r r e n t (m A )Collector-Emitter Voltage (V)Fig. 1 - Static Characteristics0100200300D C C u r r e n t G a i nCollector Current (mA)Fig. 2 - DC Current Gain Characteristics-1-0.3-100-150-100-10-500C o l l e c t o r -E m i t t e r S a t u r a t i o n V o l t a g e (m V )-10Collector Current (mA)Fig. 3 - Collector-Emitter Saturation Voltage Characteristics-1-0.2-100-150-400-800-0-1200B a s e -E m i t t e r S a t u r a t i o n V o l t a g e (m V )-10Collector Current (mA)Fig. 4 - Base-Emitter Saturation Voltage Characteristics-1-10-100-0.1-150-0.2-0.4-0.8-1.0-0.6Base-Emitter Voltage (V)C o l l c e t o r C u r r e n t (m A )Fig. 5 - Base-Emitter Voltage Characteristics02512515050100150200250C o l l e c t o r P o w e rD i s s i p a t i o n (m W )5075100Ambient Temperature (°C)Fig. 6 - Collector Power Derating CurveOrdering InformationDevice PackingPart Number-AP Ammo Packing: 20Kpcs/CartonPart Number-BP Bulk: 100Kpcs/CartonNote : Adding "-HF" Suffix for Halogen Free, eg. Part Number-TP-HF***IMPORTANT NOTICE***Micro Commercial Components Corp. reserves the right to make changes without further notice to any product herein to make corrections, modifications , enhancements , improvements , or other changes . Micro Commercial Components Corp . does not assume any liability arising out of the application or use of any product described herein; neither does it convey any license under its patent rights ,nor the rights of others . The user of products in such applications shall assume all risks of such use and will agree to hold Micro Commercial Components Corp . and all the companies whose products are represented on our website, harmless against all damages.***LIFE SUPPORT***MCC's products are not authorized for use as critical components in life support devices or systems without the expresswritten approval of Micro Commercial Components Corporation.***CUSTOMER AWARENESS***Counterfeiting of semiconductor parts is a growing problem in the industry. Micro Commercial Components (MCC) is taking strong measures to protect ourselves and our customers from the proliferation of counterfeit parts. MCC strongly encourages customers to purchase MCC parts either directly from MCC or from Authorized MCC Distributors who are listed by country on our web page cited below. Products customers buy either from MCC directly or from Authorized MCC Distributors are genuine parts, have full traceability, meet MCC's quality standards for handling and storage. MCC will not provide any warranty coverage or other assistance for parts bought from Unauthorized Sources. MCC is committed to combat this global problem and encourage our customers to do their part in stopping this practice by buying direct or from authorized distributors.。
Principles of Plasma Discharges and Materials Processing9

CHAPTER8MOLECULAR COLLISIONS8.1INTRODUCTIONBasic concepts of gas-phase collisions were introduced in Chapter3,where we described only those processes needed to model the simplest noble gas discharges: electron–atom ionization,excitation,and elastic scattering;and ion–atom elastic scattering and resonant charge transfer.In this chapter we introduce other collisional processes that are central to the description of chemically reactive discharges.These include the dissociation of molecules,the generation and destruction of negative ions,and gas-phase chemical reactions.Whereas the cross sections have been measured reasonably well for the noble gases,with measurements in reasonable agreement with theory,this is not the case for collisions in molecular gases.Hundreds of potentially significant collisional reactions must be examined in simple diatomic gas discharges such as oxygen.For feedstocks such as CF4/O2,SiH4/O2,etc.,the complexity can be overwhelming.Furthermore,even when the significant processes have been identified,most of the cross sections have been neither measured nor calculated. Hence,one must often rely on estimates based on semiempirical or semiclassical methods,or on measurements made on molecules analogous to those of interest. As might be expected,data are most readily available for simple diatomic and polyatomic gases.Principles of Plasma Discharges and Materials Processing,by M.A.Lieberman and A.J.Lichtenberg. ISBN0-471-72001-1Copyright#2005John Wiley&Sons,Inc.235236MOLECULAR COLLISIONS8.2MOLECULAR STRUCTUREThe energy levels for the electronic states of a single atom were described in Chapter3.The energy levels of molecules are more complicated for two reasons. First,molecules have additional vibrational and rotational degrees of freedom due to the motions of their nuclei,with corresponding quantized energies E v and E J. Second,the energy E e of each electronic state depends on the instantaneous con-figuration of the nuclei.For a diatomic molecule,E e depends on a single coordinate R,the spacing between the two nuclei.Since the nuclear motions are slow compared to the electronic motions,the electronic state can be determined for anyfixed spacing.We can therefore represent each quantized electronic level for a frozen set of nuclear positions as a graph of E e versus R,as shown in Figure8.1.For a mole-cule to be stable,the ground(minimum energy)electronic state must have a minimum at some value R1corresponding to the mean intermolecular separation (curve1).In this case,energy must be supplied in order to separate the atoms (R!1).An excited electronic state can either have a minimum( R2for curve2) or not(curve3).Note that R2and R1do not generally coincide.As for atoms, excited states may be short lived(unstable to electric dipole radiation)or may be metastable.Various electronic levels may tend to the same energy in the unbound (R!1)limit. Array FIGURE8.1.Potential energy curves for the electronic states of a diatomic molecule.For diatomic molecules,the electronic states are specifiedfirst by the component (in units of hÀ)L of the total orbital angular momentum along the internuclear axis, with the symbols S,P,D,and F corresponding to L¼0,+1,+2,and+3,in analogy with atomic nomenclature.All but the S states are doubly degenerate in L.For S states,þandÀsuperscripts are often used to denote whether the wave function is symmetric or antisymmetric with respect to reflection at any plane through the internuclear axis.The total electron spin angular momentum S (in units of hÀ)is also specified,with the multiplicity2Sþ1written as a prefixed superscript,as for atomic states.Finally,for homonuclear molecules(H2,N2,O2, etc.)the subscripts g or u are written to denote whether the wave function is sym-metric or antisymmetric with respect to interchange of the nuclei.In this notation, the ground states of H2and N2are both singlets,1Sþg,and that of O2is a triplet,3SÀg .For polyatomic molecules,the electronic energy levels depend on more thanone nuclear coordinate,so Figure8.1must be generalized.Furthermore,since there is generally no axis of symmetry,the states cannot be characterized by the quantum number L,and other naming conventions are used.Such states are often specified empirically through characterization of measured optical emission spectra.Typical spacings of low-lying electronic energy levels range from a few to tens of volts,as for atoms.Vibrational and Rotational MotionsUnfreezing the nuclear vibrational and rotational motions leads to additional quan-tized structure on smaller energy scales,as illustrated in Figure8.2.The simplest (harmonic oscillator)model for the vibration of diatomic molecules leads to equally spaced quantized,nondegenerate energy levelse E v¼hÀv vib vþ1 2(8:2:1)where v¼0,1,2,...is the vibrational quantum number and v vib is the linearized vibration frequency.Fitting a quadratic functione E v¼12k vib(RÀ R)2(8:2:2)near the minimum of a stable energy level curve such as those shown in Figure8.1, we can estimatev vib%k vibm Rmol1=2(8:2:3)where k vib is the“spring constant”and m Rmol is the reduced mass of the AB molecule.The spacing hÀv vib between vibrational energy levels for a low-lying8.2MOLECULAR STRUCTURE237stable electronic state is typically a few tenths of a volt.Hence for molecules in equi-librium at room temperature (0.026V),only the v ¼0level is significantly popula-ted.However,collisional processes can excite strongly nonequilibrium vibrational energy levels.We indicate by the short horizontal line segments in Figure 8.1a few of the vibrational energy levels for the stable electronic states.The length of each segment gives the range of classically allowed vibrational motions.Note that even the ground state (v ¼0)has a finite width D R 1as shown,because from(8.2.1),the v ¼0state has a nonzero vibrational energy 1h Àv vib .The actual separ-ation D R about Rfor the ground state has a Gaussian distribution,and tends toward a distribution peaked at the classical turning points for the vibrational motion as v !1.The vibrational motion becomes anharmonic and the level spa-cings tend to zero as the unbound vibrational energy is approached (E v !D E 1).FIGURE 8.2.Vibrational and rotational levels of two electronic states A and B of a molecule;the three double arrows indicate examples of transitions in the pure rotation spectrum,the rotation–vibration spectrum,and the electronic spectrum (after Herzberg,1971).238MOLECULAR COLLISIONSFor E v.D E1,the vibrational states form a continuum,corresponding to unbound classical motion of the nuclei(breakup of the molecule).For a polyatomic molecule there are many degrees of freedom for vibrational motion,leading to a very compli-cated structure for the vibrational levels.The simplest(dumbbell)model for the rotation of diatomic molecules leads to the nonuniform quantized energy levelse E J¼hÀ22I molJ(Jþ1)(8:2:4)where I mol¼m Rmol R2is the moment of inertia and J¼0,1,2,...is the rotational quantum number.The levels are degenerate,with2Jþ1states for the J th level. The spacing between rotational levels increases with J(see Figure8.2).The spacing between the lowest(J¼0to J¼1)levels typically corresponds to an energy of0.001–0.01V;hence,many low-lying levels are populated in thermal equilibrium at room temperature.Optical EmissionAn excited molecular state can decay to a lower energy state by emission of a photon or by breakup of the molecule.As shown in Figure8.2,the radiation can be emitted by a transition between electronic levels,between vibrational levels of the same electronic state,or between rotational levels of the same electronic and vibrational state;the radiation typically lies within the optical,infrared,or microwave frequency range,respectively.Electric dipole radiation is the strongest mechanism for photon emission,having typical transition times of t rad 10À9s,as obtained in (3.4.13).The selection rules for electric dipole radiation areDL¼0,+1(8:2:5a)D S¼0(8:2:5b) In addition,for transitions between S states the only allowed transitions areSþÀ!Sþand SÀÀ!SÀ(8:2:6) and for homonuclear molecules,the only allowed transitions aregÀ!u and uÀ!g(8:2:7) Hence homonuclear diatomic molecules do not have a pure vibrational or rotational spectrum.Radiative transitions between electronic levels having many different vibrational and rotational initial andfinal states give rise to a structure of emission and absorption bands within which a set of closely spaced frequencies appear.These give rise to characteristic molecular emission and absorption bands when observed8.2MOLECULAR STRUCTURE239using low-resolution optical spectrometers.As for atoms,metastable molecular states having no electric dipole transitions to lower levels also exist.These have life-times much exceeding10À6s;they can give rise to weak optical band structures due to magnetic dipole or electric quadrupole radiation.Electric dipole radiation between vibrational levels of the same electronic state is permitted for molecules having permanent dipole moments.In the harmonic oscillator approximation,the selection rule is D v¼+1;weaker transitions D v¼+2,+3,...are permitted for anharmonic vibrational motion.The preceding description of molecular structure applies to molecules having arbi-trary electronic charge.This includes neutral molecules AB,positive molecular ions ABþ,AB2þ,etc.and negative molecular ions ABÀ.The potential energy curves for the various electronic states,regardless of molecular charge,are commonly plotted on the same diagram.Figures8.3and8.4give these for some important electronic statesof HÀ2,H2,and Hþ2,and of OÀ2,O2,and Oþ2,respectively.Examples of both attractive(having a potential energy minimum)and repulsive(having no minimum)states can be seen.The vibrational levels are labeled with the quantum number v for the attrac-tive levels.The ground states of both Hþ2and Oþ2are attractive;hence these molecular ions are stable against autodissociation(ABþ!AþBþor AþþB).Similarly,the ground states of H2and O2are attractive and lie below those of Hþ2and Oþ2;hence they are stable against autodissociation and autoionization(AB!ABþþe).For some molecules,for example,diatomic argon,the ABþion is stable but the AB neutral is not stable.For all molecules,the AB ground state lies below the ABþground state and is stable against autoionization.Excited states can be attractive or repulsive.A few of the attractive states may be metastable;some examples are the 3P u state of H2and the1D g,1Sþgand3D u states of O2.Negative IonsRecall from Section7.2that many neutral atoms have a positive electron affinity E aff;that is,the reactionAþeÀ!AÀis exothermic with energy E aff(in volts).If E aff is negative,then AÀis unstable to autodetachment,AÀ!Aþe.A similar phenomenon is found for negative molecular ions.A stable ABÀion exists if its ground(lowest energy)state has a potential minimum that lies below the ground state of AB.This is generally true only for strongly electronegative gases having large electron affinities,such as O2 (E aff%1:463V for O atoms)and the halogens(E aff.3V for the atoms).For example,Figure8.4shows that the2P g ground state of OÀ2is stable,with E aff% 0:43V for O2.For weakly electronegative or for electropositive gases,the minimum of the ground state of ABÀgenerally lies above the ground state of AB,and ABÀis unstable to autodetachment.An example is hydrogen,which is weakly electronegative(E aff%0:754V for H atoms).Figure8.3shows that the2Sþu ground state of HÀ2is unstable,although the HÀion itself is stable.In an elec-tropositive gas such as N2(E aff.0),both NÀ2and NÀare unstable. 240MOLECULAR COLLISIONS8.3ELECTRON COLLISIONS WITH MOLECULESThe interaction time for the collision of a typical (1–10V)electron with a molecule is short,t c 2a 0=v e 10À16–10À15s,compared to the typical time for a molecule to vibrate,t vib 10À14–10À13s.Hence for electron collisional excitation of a mole-cule to an excited electronic state,the new vibrational (and rotational)state canbeFIGURE 8.3.Potential energy curves for H À2,H 2,and H þ2.(From Jeffery I.Steinfeld,Molecules and Radiation:An Introduction to Modern Molecular Spectroscopy ,2d ed.#MIT Press,1985.)8.3ELECTRON COLLISIONS WITH MOLECULES 241FIGURE 8.4.Potential energy curves for O À2,O 2,and O þ2.(From Jeffery I.Steinfeld,Molecules and Radiation:An Introduction to Modern Molecular Spectroscopy ,2d ed.#MIT Press,1985.)242MOLECULAR COLLISIONS8.3ELECTRON COLLISIONS WITH MOLECULES243 determined by freezing the nuclear motions during the collision.This is known as the Franck–Condon principle and is illustrated in Figure8.1by the vertical line a,showing the collisional excitation atfixed R to a high quantum number bound vibrational state and by the vertical line b,showing excitation atfixed R to a vibra-tionally unbound state,in which breakup of the molecule is energetically permitted. Since the typical transition time for electric dipole radiation(t rad 10À9–10À8s)is long compared to the dissociation( vibrational)time t diss,excitation to an excited state will generally lead to dissociation when it is energetically permitted.Finally, we note that the time between collisions t c)t rad in typical low-pressure processing discharges.Summarizing the ordering of timescales for electron–molecule collisions,we havet at t c(t vib t diss(t rad(t cDissociationElectron impact dissociation,eþABÀ!AþBþeof feedstock gases plays a central role in the chemistry of low-pressure reactive discharges.The variety of possible dissociation processes is illustrated in Figure8.5.In collisions a or a0,the v¼0ground state of AB is excited to a repulsive state of AB.The required threshold energy E thr is E a for collision a and E a0for Array FIGURE8.5.Illustrating the variety of dissociation processes for electron collisions with molecules.collision a0,and it leads to an energy after dissociation lying between E aÀE diss and E a0ÀE diss that is shared among the dissociation products(here,A and B). Typically,E aÀE diss few volts;consequently,hot neutral fragments are typically generated by dissociation processes.If these hot fragments hit the substrate surface, they can profoundly affect the process chemistry.In collision b,the ground state AB is excited to an attractive state of AB at an energy E b that exceeds the binding energy E diss of the AB molecule,resulting in dissociation of AB with frag-ment energy E bÀE diss.In collision b0,the excitation energy E b0¼E diss,and the fragments have low energies;hence this process creates fragments having energies ranging from essentially thermal energies up to E bÀE diss few volts.In collision c,the AB atom is excited to the bound excited state ABÃ(labeled5),which sub-sequently radiates to the unbound AB state(labeled3),which then dissociates.The threshold energy required is large,and the fragments are hot.Collision c can also lead to dissociation of an excited state by a radiationless transfer from state5to state4near the point where the two states cross:ABÃðboundÞÀ!ABÃðunboundÞÀ!AþBÃThe fragments can be both hot and in excited states.We discuss such radiationless electronic transitions in the next section.This phenomenon is known as predisso-ciation.Finally,a collision(not labeled in thefigure)to state4can lead to dis-sociation of ABÃ,again resulting in hot excited fragments.The process of electron impact excitation of a molecule is similar to that of an atom,and,consequently,the cross sections have a similar form.A simple classical estimate of the dissociation cross section for a level having excitation energy U1can be found by requiring that an incident electron having energy W transfer an energy W L lying between U1and U2to a valence electron.Here,U2is the energy of the next higher level.Then integrating the differential cross section d s[given in(3.4.20)and repeated here],d s¼pe24021Wd W LW2L(3:4:20)over W L,we obtains diss¼0W,U1pe24pe021W1U1À1WU1,W,U2pe24021W1U1À1U2W.U28>>>>>><>>>>>>:(8:3:1)244MOLECULAR COLLISIONSLetting U2ÀU1(U1and introducing voltage units W¼e E,U1¼e E1and U2¼e E2,we haves diss¼0E,E1s0EÀE11E1,E,E2s0E2ÀE1EE.E28>>>><>>>>:(8:3:2)wheres0¼pe4pe0E12(8:3:3)We see that the dissociation cross section rises linearly from the threshold energy E thr%E1to a maximum value s0(E2ÀE1)=E thr at E2and then falls off as1=E. Actually,E1and E2can depend on the nuclear separation R.In this case,(8.3.2) should be averaged over the range of R s corresponding to the ground-state vibrational energy,leading to a broadened dependence of the average cross section on energy E.The maximum cross section is typically of order10À15cm2. Typical rate constants for a single dissociation process with E thr&T e have an Arrhenius formK diss/K diss0expÀE thr T e(8:3:4)where K diss0 10À7cm3=s.However,in some cases E thr.T e.For excitation to an attractive state,an appropriate average over the fraction of the ground-state vibration that leads to dissociation must be taken.Dissociative IonizationIn addition to normal ionization,eþABÀ!ABþþ2eelectron–molecule collisions can lead to dissociative ionizationeþABÀ!AþBþþ2eThese processes,common for polyatomic molecules,are illustrated in Figure8.6.In collision a having threshold energy E iz,the molecular ion ABþis formed.Collisionsb andc occur at higher threshold energies E diz and result in dissociative ionization,8.3ELECTRON COLLISIONS WITH MOLECULES245leading to the formation of fast,positively charged ions and neutrals.These cross sections have a similar form to the Thompson ionization cross section for atoms.Dissociative RecombinationThe electron collision,e þAB þÀ!A þB Ãillustrated as d and d 0in Figure 8.6,destroys an electron–ion pair and leads to the production of fast excited neutral fragments.Since the electron is captured,it is not available to carry away a part of the reaction energy.Consequently,the collision cross section has a resonant character,falling to very low values for E ,E d and E .E d 0.However,a large number of excited states A Ãand B Ãhaving increasing principal quantum numbers n and energies can be among the reaction products.Consequently,the rate constants can be large,of order 10À7–10À6cm 3=s.Dissocia-tive recombination to the ground states of A and B cannot occur because the potential energy curve for AB þis always greater than the potential energycurveFIGURE 8.6.Illustration of dissociative ionization and dissociative recombination for electron collisions with molecules.246MOLECULAR COLLISIONSfor the repulsive state of AB.Two-body recombination for atomic ions or for mol-ecular ions that do not subsequently dissociate can only occur with emission of a photon:eþAþÀ!Aþh n:As shown in Section9.2,the rate constants are typically three tofive orders of magnitude lower than for dissociative recombination.Example of HydrogenThe example of H2illustrates some of the inelastic electron collision phenomena we have discussed.In order of increasing electron impact energy,at a threshold energy of 8:8V,there is excitation to the repulsive3Sþu state followed by dissociation into two fast H fragments carrying 2:2V/atom.At11.5V,the1Sþu bound state is excited,with subsequent electric dipole radiation in the ultraviolet region to the1Sþg ground state.At11.8V,there is excitation to the3Sþg bound state,followedby electric dipole radiation to the3Sþu repulsive state,followed by dissociation with 2:2V/atom.At12.6V,the1P u bound state is excited,with UV emission tothe ground state.At15.4V,the2Sþg ground state of Hþ2is excited,leading to the pro-duction of Hþ2ions.At28V,excitation of the repulsive2Sþu state of Hþ2leads to thedissociative ionization of H2,with 5V each for the H and Hþfragments.Dissociative Electron AttachmentThe processes,eþABÀ!AþBÀproduce negative ion fragments as well as neutrals.They are important in discharges containing atoms having positive electron affinities,not only because of the pro-duction of negative ions,but because the threshold energy for production of negative ion fragments is usually lower than for pure dissociation processes.A variety of pro-cesses are possible,as shown in Figure8.7.Since the impacting electron is captured and is not available to carry excess collision energy away,dissociative attachment is a resonant process that is important only within a narrow energy range.The maximum cross sections are generally much smaller than the hard-sphere cross section of the molecule.Attachment generally proceeds by collisional excitation from the ground AB state to a repulsive ABÀstate,which subsequently either auto-detaches or dissociates.The attachment cross section is determined by the balance between these processes.For most molecules,the dissociation energy E diss of AB is greater than the electron affinity E affB of B,leading to the potential energy curves shown in Figure8.7a.In this case,the cross section is large only for impact energies lying between a minimum value E thr,for collision a,and a maximum value E0thr for8.3ELECTRON COLLISIONS WITH MOLECULES247FIGURE 8.7.Illustration of a variety of electron attachment processes for electron collisions with molecules:(a )capture into a repulsive state;(b )capture into an attractive state;(c )capture of slow electrons into a repulsive state;(d )polar dissociation.248MOLECULAR COLLISIONScollision a 0.The fragments are hot,having energies lying between minimum and maximum values E min ¼E thr þE affB ÀE diss and E max ¼E 0thr þE af fB ÀE diss .Since the AB Àstate lies above the AB state for R ,R x ,autodetachment can occur as the mol-ecules begin to separate:AB À!AB þe.Hence the cross section for production of negative ions can be much smaller than that for excitation of the AB Àrepulsive state.As a crude estimate,for the same energy,the autodetachment rate is ffiffiffiffiffiffiffiffiffiffiffiffiffiM R =m p 100times the dissociation rate of the repulsive AB Àmolecule,where M R is the reduced mass.Hence only one out of 100excitations lead to dissociative attachment.Excitation to the AB Àbound state can also lead to dissociative attachment,as shown in Figure 8.7b .Here the cross section is significant only for E thr ,E ,E 0thr ,but the fragments can have low energies,with a minimum energy of zero and a maximum energy of E 0thr þE affB ÀE diss .Collision b,e þAB À!AB ÀÃdoes not lead to production of AB Àions because energy and momentum are not gen-erally conserved when two bodies collide elastically to form one body (see Problem3.12).Hence the excited AB ÀÃion separates,AB ÀÃÀ!e þABunless vibrational radiation or collision with a third body carries off the excess energy.These processes are both slow in low-pressure discharges (see Section 9.2).At high pressures (say,atmospheric),three-body attachment to form AB Àcan be very important.For a few molecules,such as some halogens,the electron affinity of the atom exceeds the dissociation energy of the neutral molecule,leading to the potential energy curves shown in Figure 8.7c .In this case the range of electron impact ener-gies E for excitation of the AB Àrepulsive state includes E ¼0.Consequently,there is no threshold energy,and very slow electrons can produce dissociative attachment,resulting in hot neutral and negative ion fragments.The range of R s over which auto-detachment can occur is small;hence the maximum cross sections for dissociative attachment can be as high as 10À16cm 2.A simple classical estimate of electron capture can be made using the differential scattering cross section for energy loss (3.4.20),in a manner similar to that done for dissociation.For electron capture to an energy level E 1that is unstable to autode-tachment,and with the additional constraint for capture that the incident electron energy lie within E 1and E 2¼E 1þD E ,where D E is a small energy difference characteristic of the dissociative attachment timescale,we obtain,in place of (8.3.2),s att¼0E ,E 1s 0E ÀE 1E 1E 1,E ,E 20E .E 28>><>>:(8:3:5)8.3ELECTRON COLLISIONS WITH MOLECULES 249wheres 0%p m M R 1=2e 4pe 0E 1 2(8:3:6)The factor of (m =M R )1=2roughly gives the fraction of excited states that do not auto-detach.We see that the dissociative attachment cross section rises linearly at E 1to a maximum value s 0D E =E 1and then falls abruptly to zero.As for dissociation,E 1can depend strongly on the nuclear separation R ,and (8.3.5)must be averaged over the range of E 1s corresponding to the ground state vibrational motion;e.g.,from E thr to E 0thr in Figure 8.7a .Because generally D E (E 0thr ÀE thr ,we can write (8.3.5)in the forms att %p m M R 1=2e 4pe 0 2(D E )22E 1d (E ÀE 1)(8:3:7)where d is the Dirac delta ing (8.3.7),the average over the vibrational motion can be performed,leading to a cross section that is strongly peaked lying between E thr and E 0thr .We leave the details of the calculation to a problem.Polar DissociationThe process,e þAB À!A þþB Àþeproduces negative ions without electron capture.As shown in Figure 8.7d ,the process proceeds by excitation of a polar state A þand B Àof AB Ãthat has a separ-ated atom limit of A þand B À.Hence at large R ,this state lies above the A þB ground state by the difference between the ionization potential of A and the electron affinity of B.The polar state is weakly bound at large R by the Coulomb attraction force,but is repulsive at small R .The maximum cross section and the dependence of the cross section on electron impact energy are similar to that of pure dissociation.The threshold energy E thr for polar dissociation is generally large.The measured cross section for negative ion production by electron impact in O 2is shown in Figure 8.8.The sharp peak at 6.5V is due to dissociative attachment.The variation of the cross section with energy is typical of a resonant capture process.The maximum cross section of 10À18cm 2is quite low because autode-tachment from the repulsive O À2state is strong,inhibiting dissociative attachment.The second gradual maximum near 35V is due to polar dissociation;the variation of the cross section with energy is typical of a nonresonant process.250MOLECULAR COLLISIONS。
多孔材料吸附重金属英文

Porous Materials for Heavy Metal AdsorptionIntroductionPorous materials have gained significant attention in environmental science and technology due to their high surface area and unique structural properties. They possess interconnected networks of pores, which make them excellent candidates for various applications, including adsorption of heavy metals. Heavy metals, such as lead (Pb), cadmium (Cd), mercury (Hg), and arsenic (As), are highly toxic and can pose serious risks to human health and the environment. Therefore, the development of effective adsorbents for heavy metal removal is of utmost importance. In this article, we will discuss the characteristics of porous materials and their applications in heavy metal adsorption.Characteristics of Porous MaterialsPorous materials are solid substances that have internal pores or cavities. These pores can be categorized into three types based on their sizes: micropores (less than 2 nm), mesopores (2-50 nm), and macropores (larger than 50 nm). The presence of these pores provides a large surface area, which facilitates the adsorption of heavy metal ions. Additionally, the surface chemistry and functional groups of porous materials can be tailored to enhance their adsorption capacity.Types of Porous Materials1.Activated Carbon (AC): Activated carbon is a widely used porousmaterial due to its excellent adsorption properties. It is derived from various carbonaceous sources and possesses a highly porous structure with a largespecific surface area. The adsorption of heavy metal ions on activated carbon occurs through various mechanisms, such as ion exchange, electrostaticattraction, and complex formation.2.Metal-Organic Frameworks (MOFs): MOFs are a class of porousmaterials composed of metal ions or clusters coordinated with organic ligands.They exhibit high porosity, diverse structures, and tunable pore sizes, making them promising candidates for heavy metal adsorption. The metal centers in MOFs can interact with heavy metal ions through coordination bonds, enabling efficient removal.3.Mesoporous Silica: Mesoporous silica materials, such as SBA-15 andMCM-41, have ordered porous structures with uniform pore sizes. Theypossess high surface areas and large pore volumes, making them suitable for heavy metal adsorption. The surface of mesoporous silica can be functionalized with different organic groups to enhance the specific adsorption of heavy metal ions.Applications of Porous Materials in Heavy Metal Adsorption1.Water Treatment: Porous materials, such as activated carbon, arewidely used in water treatment processes to remove heavy metal ions. These materials can be employed in fixed-bed columns, batch experiments, ormembrane systems to effectively adsorb heavy metals from contaminatedwater sources.2.Industrial Effluent Treatment: Various industries generate effluentscontaining heavy metals, which need to be treated before discharge. Porousmaterials offer a cost-effective and efficient solution for the removal of heavy metal contaminants from industrial wastewaters.3.Soil Remediation: Porous materials can be used for soil remediationby immobilizing heavy metal ions, preventing their leaching into groundwater.The materials can be applied through in-situ injection or incorporation into soil amendments, allowing for the effective remediation of contaminated soils.ConclusionPorous materials possess unique structural characteristics and surface properties, which make them ideal for heavy metal adsorption. Activated carbon, metal-organic frameworks, and mesoporous silica are commonly used porous materials in heavy metal removal applications. Their high surface area, tunable pore sizes, and functionalizable surfaces enable efficient adsorption of heavy metal ions. Porous materials find applications in water treatment, industrial effluent treatment, and soil remediation. Continued research and development in porous materials for heavy metal adsorption will contribute to addressing the ongoing challenges associated with heavy metal pollution.。
First-principles study of the structural, vibrational, phonon and thermodynamic
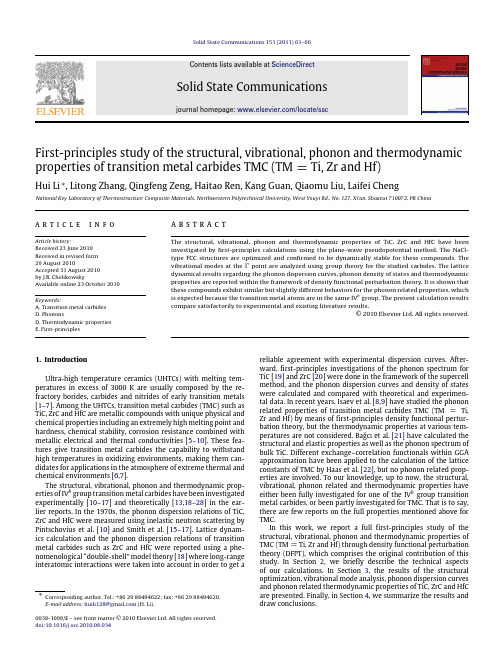
1. Introduction Ultra-high temperature ceramics (UHTCs) with melting temperatures in excess of 3000 K are usually composed by the refractory borides, carbides and nitrides of early transition metals [1–7]. Among the UHTCs, transition metal carbides (TMC) such as TiC, ZrC and HfC are metallic compounds with unique physical and chemical properties including an extremely high melting point and hardness, chemical stability, corrosion resistance combined with metallic electrical and thermal conductivities [5–10]. These features give transition metal carbides the capability to withstand high temperatures in oxidizing environments, making them candidates for applications in the atmosphere of extreme thermal and chemical environments [6,7]. The structural, vibrational, phonon and thermodynamic properties of IVb group transition metal carbides have been investigated experimentally [10–17] and theoretically [13,18–28] in the earlier reports. In the 1970s, the phonon dispersion relations of TiC, ZrC and HfC were measured using inelastic neutron scattering by Pintschovius et al. [10] and Smith et al. [15–17]. Lattice dynamics calculation and the phonon dispersion relations of transition metal carbides such as ZrC and HfC were reported using a phenomenological ‘‘double-shell’’ model theory [18] where long-range interatomic interactions were taken into account in order to get a
langchain 调用质谱
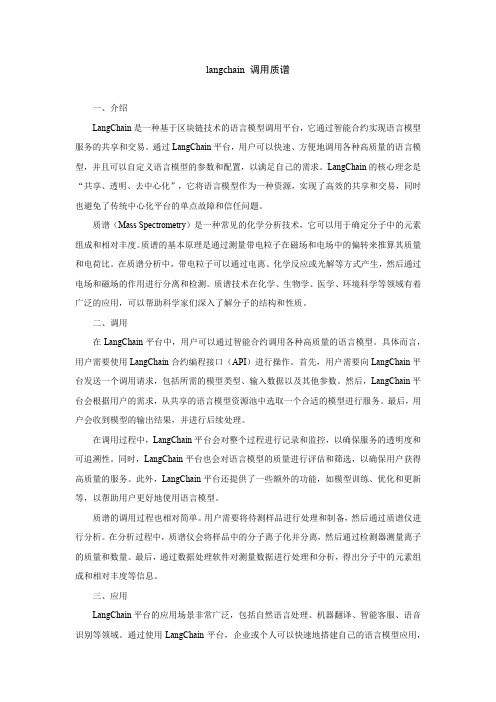
langchain 调用质谱一、介绍LangChain是一种基于区块链技术的语言模型调用平台,它通过智能合约实现语言模型服务的共享和交易。
通过LangChain平台,用户可以快速、方便地调用各种高质量的语言模型,并且可以自定义语言模型的参数和配置,以满足自己的需求。
LangChain的核心理念是“共享、透明、去中心化”,它将语言模型作为一种资源,实现了高效的共享和交易,同时也避免了传统中心化平台的单点故障和信任问题。
质谱(Mass Spectrometry)是一种常见的化学分析技术,它可以用于确定分子中的元素组成和相对丰度。
质谱的基本原理是通过测量带电粒子在磁场和电场中的偏转来推算其质量和电荷比。
在质谱分析中,带电粒子可以通过电离、化学反应或光解等方式产生,然后通过电场和磁场的作用进行分离和检测。
质谱技术在化学、生物学、医学、环境科学等领域有着广泛的应用,可以帮助科学家们深入了解分子的结构和性质。
二、调用在LangChain平台中,用户可以通过智能合约调用各种高质量的语言模型。
具体而言,用户需要使用LangChain合约编程接口(API)进行操作。
首先,用户需要向LangChain平台发送一个调用请求,包括所需的模型类型、输入数据以及其他参数。
然后,LangChain平台会根据用户的需求,从共享的语言模型资源池中选取一个合适的模型进行服务。
最后,用户会收到模型的输出结果,并进行后续处理。
在调用过程中,LangChain平台会对整个过程进行记录和监控,以确保服务的透明度和可追溯性。
同时,LangChain平台也会对语言模型的质量进行评估和筛选,以确保用户获得高质量的服务。
此外,LangChain平台还提供了一些额外的功能,如模型训练、优化和更新等,以帮助用户更好地使用语言模型。
质谱的调用过程也相对简单。
用户需要将待测样品进行处理和制备,然后通过质谱仪进行分析。
在分析过程中,质谱仪会将样品中的分子离子化并分离,然后通过检测器测量离子的质量和数量。
CIRC E-PIT
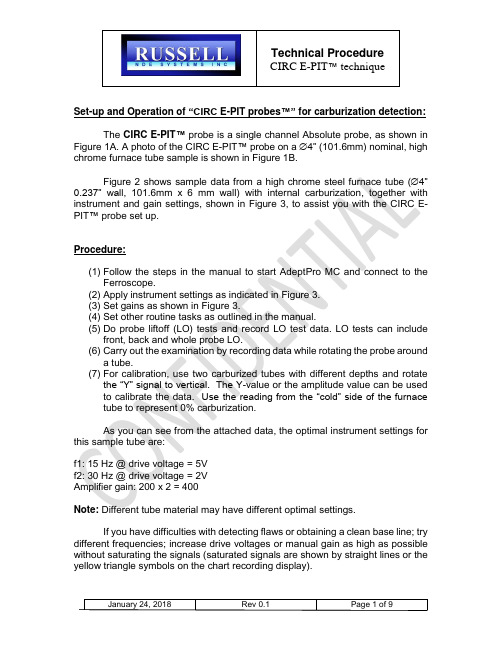
Set-up and Operation of “CIRC E-PIT probes™” for carburization detection:The CIRC E-PIT™ probe is a single channel Absolute probe, as shown in Figure 1A. A photo of the CIRC E-PIT™ probe on a ∅4” (101.6mm) nominal, high chrome furnace tube sample is shown in Figure 1B.Figure 2 shows sample data from a high chrome steel furnace tube (∅4”0.237” wall, 101.6mm x 6 mm wall) with internal carburization, together with instrument and gain settings, shown in Figure 3, to assist you with the CIRC E-PIT™ probe set up.Procedure:(1) Follow the steps in the manual to start AdeptPro MC and connect to theFerroscope.(2) Apply instrument settings as indicated in Figure 3.(3) Set gains as shown in Figure 3.(4) Set other routine tasks as outlined in the manual.(5) Do probe liftoff (LO) tests and record LO test data. LO tests can includefront, back and whole probe LO.(6) Carry out the examination by recording data while rotating the probe arounda tube.(7) For calibration, use two carburized tubes with different depths and rotatethe “Y” signal to vertical. The Y-value or the amplitude value can be used to calibrate the data. Use the reading from the “cold” side of the furnace tube to represent 0% carburization.As you can see from the attached data, the optimal instrument settings for this sample tube are:f1: 15 Hz @ drive voltage = 5Vf2: 30 Hz @ drive voltage = 2VAmplifier gain: 200 x 2 = 400Note: Different tube material may have different optimal settings.If you have difficulties with detecting flaws or obtaining a clean base line; try different frequencies; increase drive voltages or manual gain as high as possible without saturating the signals (saturated signals are shown by straight lines or the yellow triangle symbols on the chart recording display).Figure 1A. CIRC E-PIT™ Coils layoutFigure 1B. CIRC E-PIT™ photoThis procedure will detect and quantify carburization of furnace tubes that starts on the inside of the tube.Carburization affects furnace tubes in a variety of process environments, and embrittles the tube over time, leading to eventual brittle failure.The carburization process requires furnace temperatures generally above 800 degrees-C and a source of carbon. In the examples shown below, the tube was in a coker furnace service, with heavy oil flowing on the inside of the high-chrome tube, and a heat source (burning natural gas) on the outside of the tube.In this example, the source of carbon is the heavy oil, which deposits a thin layer of coke on the hot side of the tube. The coke provides the carbon source which slowly permeates into the tube wall, making it harder and more brittle.The reason that this remote-field method is so effective at detecting the depth of carburization is because carburization strongly affects the electromagnetic properties of the tube. One way to find the areas in a furnace that are more carburized that others is to use a regular E-PIT™, longitudinal scanning probe first, and then use the CIRC E-PIT™ to measure the maximum depth of carburization in those areas.Figure-4: example of ID carburized tube (Nital etched)Note: carburized material appears as a lighter colourFigure-5 showing internal coke layer and 2mm deep ID carburized case Note: carburized material is a lighter colourFigure-6: 0.8mm ID carburized case thickness with very little internal coke Note: Carburized material is a lighter colourFigure-7: 2.0mm ID case depth with thick coke deposit and light ID pitting Note: Carburized material is a lighter colourNotes:1. in all examples shown, the maximum carburization was on the hot side ofthe tube (closest or facing the gas burners.2. Carburization can also start from the outside of the tube, acquiring itscarbon from the combustion fuel. This process is typically slower andaffects the cold side of the tube as well (i.e. the tube may be carburized at all points on the circumference, depending on the elevation of the tube inthe furnace…which assumes that higher elevations are hotter).3. The CIRC E-PIT probe is designed so that it can get very close toattachments such as hangars. This allows the operator to check if theattachment has acted as a concentrator to the heat, thereby locallyoverheating the tube and accelerating the carburization.4. For internal carburization, phase can be used to predict case depth. In ourexample above, there is about 0.3 degrees of phase change for each 1%of case depth increase. However, for external carburization, phase cannot be used as there is no phase change for varying depths of carburization.In this case, Amplitude or Y-component can be used.5. In both cases, calibration on samples of known carburization depths isessential.Figure-8: external carburization example.Note: Carburized material is a lighter colourNotes:1) In the example of external carburization shown in Figure-8, note that thecarburized material (lighter colour) is 100% through wall in the centre ofthe photo and decreases to 50% and then 30% as the distance from thehot side increases.2) The 100% through-wall carburization was in an area where there was anattachment (hangar)3) This type of localized, through-wall carburization has caused furnace tubefailure and fires on unit start-up。
- 1、下载文档前请自行甄别文档内容的完整性,平台不提供额外的编辑、内容补充、找答案等附加服务。
- 2、"仅部分预览"的文档,不可在线预览部分如存在完整性等问题,可反馈申请退款(可完整预览的文档不适用该条件!)。
- 3、如文档侵犯您的权益,请联系客服反馈,我们会尽快为您处理(人工客服工作时间:9:00-18:30)。
a r X i v :n u c l -e x /0411034v 1 18 N o v 20041Probing bulk properties and partonic collectivity via multi-strange baryons in Au+Au collisions at top RHIC energy.Magali Estiennea(for the STAR Collaboration)aSUBATECH,4rue Alfred Kastler,BP 20722,44307Nantes-cedex 3,FRANCEThe study of the multi-strange baryon production in the final state of ultra-relativisticAu+Au collisions at√Ξ+and Ω−+2suggesting an expansion and the cooling of the system between chemical and thermal FO.Concerning multi-strange baryons,it has been suggested that these particles should not develop such significant transverse radial flow due to their presumably small cross section so that they should decouple much earlier in the collision [6,7].Their observed transverse radialflow would then primarily reflects partonic flow behaviour.Elliptic flow due to the initial asymmetry of the system in non-central collisions has also proven to be a good tool for understanding the properties of the early stage of the collisions [8].Thus multi-strange baryon elliptic flow could be a valuable probe of the initial partonic system.As flow is an additive quantity,we present both radial transverse flow and elliptic flow measurements of multi-strange baryons in order to disentangle its hadronic and partonic contributions.2.RESULTS AND DISCUSSION 2.1.Bulk chemical propertiesAll the data presented in this contri-ch pancy factor γs (bottom)as a function of the number of participants.T ch and γs have been calculated from statistical fits includingπ,K ,p (squares)and π,K ,p ,Λ,Φ,Ξ,Ωparticles (circles).bution have been collected by the STARdetector described in [9].Multi-strange particles are identified via the topology oftheir decay Ξ→Λ+πand Ω→Λ+K thenthe subsequent decay Λ→p +πwith the re-spective branching ratios 100%,68%and 64%.For more details see [10].Correc-tions for tracking efficiency and detectoracceptance were applied.The final cor-rected transverse momentum distributionsare fitted in order to extract yields and in-verse slope parameters.The results of a statistical fit of particleratios including π±,K ±,p ,Λ,Φ,Ξ±and Ω±are presented on Figure 1.Verygood agreement between our data and themodel are achieved for each centrality at √p .For the most central collisions,the four free parameters of the fit are T ch =157±6MeV ,µB =22±4MeV ,µs =3.8±2.6MeV and γs =0.86±0.11.The evolution of T ch and γs with centrality arerepresented as square symbols on Figure 1.An other fit has also been performed including then all the hadrons π±,K ±,p ,Λ,Φ,Ξ±and Ω±.The parameters obtained for the most central collision are T ch =160±5MeV ,µB =24±4MeV ,µs =1.4±1.6MeV and γs =0.99±0.07.T ch and γs evolutions with centrality are represented as circles.We note no dependence of T ch with centrality.All the particles seem to chemically freeze-out at a3 temperature of160±5MeV close to LQCD predictions,T ch seems to be essentiallyfixed by the most numerousπ,K,p particles and does not seem to be dependent on the initial system size.From peripheral to central collisions,including(multi-)strange hadrons in thefit,γs increases from0.8and saturates at1.This value suggests that in most central collisions at top RHIC energy,the phase space is saturated in strange quarks so that the system is close to strangeness equilibration.This increase ofγs also signs the existence of significant sΞ+have been studied.ForΩ−+4indicate that Au+Au collisions with different initial conditions evolve always to the same chemical FO temperature,and then cool down further to a kinetic FO dependent on centrality.So this radialflow scenario suggests that for multi-strange baryons,a significant fraction (if not all)of the transverseflow has been developed probably in a partonic phase of the system so that multi-strange baryons should develop ellipticflow.Figure3shows the measurement of the ellipticflow v2ofΞ−+¯Ξ+andΩ−+¯Ω+as a function of p T for the minimum bias data.v2of K0s andΛ+¯Λpreviously measured[12]are also represented for comparison.2s¯Ξ+andΩ−+¯Ω+from200GeV Au+Au min-imum bias collisions.Hydrodynamic modelcalculations are shown(colored zone)as wellas hydro-inspired model calculations(thicklines)but are not commented in this letter[13].Figure4.Ellipticflow v2of K0s,Λ+¯ΛandΞ−+¯Ξ+normalized to the numberof constituent quarks(n)as a function ofp T/n.First we observe that v2of multi-strange baryons is different from zero and seems to follow the same behaviour asΛv2.That means same shape(saturation at a p T∼3GeV/c) and same amplitude(saturation at v2∼20%).In the low p T region,Ξv2is in agreement with hydrodynamic model calculations(colored zone)which predict its mass ordering in this p T region.However,for a p T>2GeV/c,v2deviates from Hydrodynamic model prediction and shows different behaviour for K0s which saturates at a p T=2GeV/c, at a value around14%compared to the strange baryons v2.It confirms a previously established baryon to meson dependence of the ellipticflow parameter from a particle mass dependence in the intermediate p T region[12].This particle type dependence is well and“simply”explained by quark coalescence or recombination models[14,15]in which hadrons are dominantly produced by the coalescence of constituent quarks from a partonic system supporting the idea of a collectivity between partons.These models predict a universal scaling of transverse momentum p T and ellipticflow to the number of constituent quarks(n).Previously,such scaling has been demonstrated for the mesons K0s and the baryonsΛat intermediate p T[12].Figure4shows the superposition of the5 scaled ellipticflows v2/n=f(p T)/n for K0s,Λas well as forΞ−+。