Magnetic Structures of High Temperature Phases of TbBaCo2O5.5
聚合物基四氧化三铁磁性度材料的制备
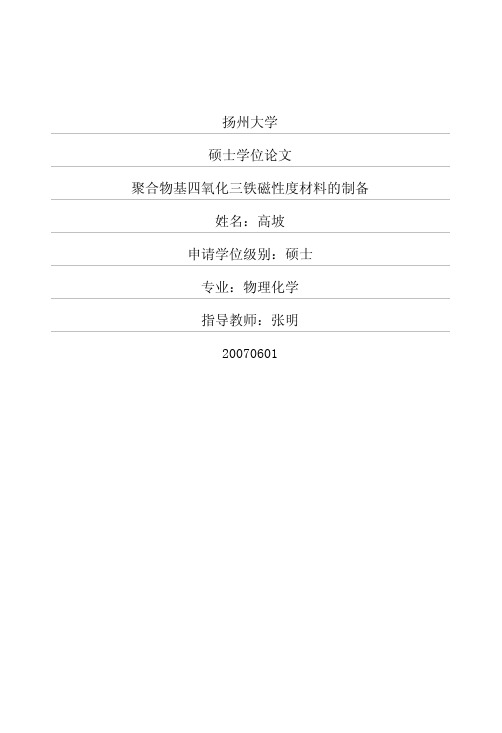
扬州大学硕士学位论文聚合物基四氧化三铁磁性度材料的制备姓名:高坡申请学位级别:硕士专业:物理化学指导教师:张明200706010扬州大学顾}学位论文及纤维管束构成的天然梯度材料。
自然界中的这种功能梯度材料是生物在适应周围环境优胜劣汰的进化中采取的一种最优化的材料构成方式。
所谓功能梯度材料(FunctionallyGradedMaterial简称FGM)就是由一种全新的设计概念而开发的新型功能材料【4】。
功能梯度材料是在材料的制备过程中,选择两种或两种以上性质不同的材料,通过连续地控制材料的微观要素(包括组成和结构),使其沿某一方向由一侧向另一侧呈连续性变化或非连续性变化,从而使材料的性质和功能也呈梯度变化的新型材料pJ。
幽1.1功能梯度材料的梯度方向Fi91.1Gradeddirectionoffunctionallygraded图1,2SiC/C功能梯度材料的断面图Fi91.2Electronmicrographsofofthecross・sectionofas—prepared当前对功能梯度材料的分类尚没有统一的标准。
根掘不同的梯度性质变化,功能梯度材料可分为密度功能梯度材料、成分功能梯度材料、光学功能梯度材料和精细功能梯度材料等。
根据不同的应用领域,功能梯度材料可分为耐热功能梯度材料、生物功能梯度材料、化学工程功能梯度材料、磁性功能梯度材料和电子工程功能梯度材料等。
根据不同的结构,功能梯度材料可分为连续性功能梯度材料[(见平面图1.1a),如图1.2为SiC/C【6】连续性功能梯度材料的电子显微镜图]高坡聚合物摹旧瓴化.三铁磁惮梯度村料的制备和非连续性功能梯度材料[(见截面图1.1b.c-d),如图1.3为W/Cu[71的非连续性功能梯度材料]:图1.3W/Cu功能梯度材料的显微镜图Fi91.3Micrographofwell—sinteredW/CuFGM表1FGM制备的典型方法TableltypicalpreparationmethodofFGM高坡聚合物基叫氧化三铁磁性梯度材料的制各41图2.5是在常温下,利用水相共沉淀法制备出来的磁性四氧化三铁纳米粒子的磁滞回线图,由图可得磁性四氧化三铁纳米粒子的比饱和磁化强度or=56.58emu/g,可知制备的粒子具有较强的磁性,且具有超顺磁性。
热处理-铁碳相图-Fe3C-奥氏体,马氏体,铁素体参考幻灯片
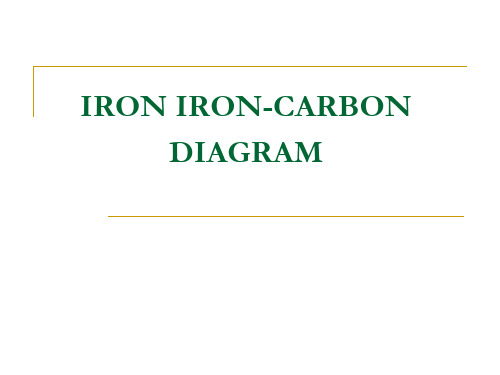
Definition of structures
Pearlite is the eutectoid mixture containing 0.80 % C and is formed at 723°C on very slow cooling.
It is a very fine platelike or lamellar mixture of ferrite and cementite.
IRON IRON-CARBON DIAGRAM
IRON IRON-CARBON DIAGRAM
eutectoid
Eutectic
Pearlite and Cementine
Austenite
Ferrite Pearlite
Pearlite and Carbide
Steel
Cast iron
Outline
Definition of structures
Ferrite
Average properties are:
Tensile strength = 40,000 psi;
Elongation
= 40 % in 2 in;
Hardness
> Rockwell C 0 or > Rockwell B 90
The white ferritic background or matrix contains thin plates of cementite (dark).
Definition of structures
Pearlite
Average properties are:
Tensile strength = 120,000 psi;
brittle intermetallic compound of iron & carbon, as Fe3C, contains 6.67 % C. It is the hardest structure that appears on the diagram, exact melting point unknown. Its crystal structure is orthorhombic. It is has low tensile strength (approx. 5,000 psi),
纳米材料与微型机器外文文献翻译、中英文翻译
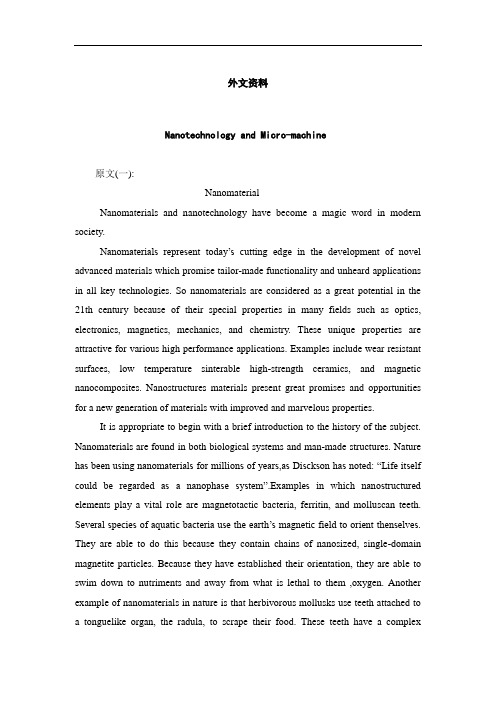
外文资料Nanotechnology and Micro-machine原文(一):NanomaterialNanomaterials and nanotechnology have become a magic word in modern society.Nanomaterials represent today’s cutting edge in the development of novel advanced materials which promise tailor-made functionality and unheard applications in all key technologies. So nanomaterials are considered as a great potential in the 21th century because of their special properties in many fields such as optics, electronics, magnetics, mechanics, and chemistry. These unique properties are attractive for various high performance applications. Examples include wear resistant surfaces, low temperature sinterable high-strength ceramics, and magnetic nanocomposites. Nanostructures materials present great promises and opportunities for a new generation of materials with improved and marvelous properties.It is appropriate to begin with a brief introduction to the history of the subject. Nanomaterials are found in both biological systems and man-made structures. Nature has been using nanomaterials for millions of years,as Disckson has noted: “Life itself could be regarded as a nanophase system”.Examples in which nanostructured elements play a vital role are magnetotactic bacteria, ferritin, and molluscan teeth. Several species of aquatic bacteria use the earth’s magnetic field to orient thenselves. They are able to do this because they contain chains of nanosized, single-domain magnetite particles. Because they have established their orientation, they are able to swim down to nutriments and away from what is lethal to them ,oxygen. Another example of nanomaterials in nature is that herbivorous mollusks use teeth attached to a tonguelike organ, the radula, to scrape their food. These teeth have a complexstructure containing nanocrystalline needles. We can utilize biological templates formaking nanomaterials. Apoferritin has been used as a confined reaction environmentfor the synthesis of nanosized magnetite particles. Some scholars consider biologicalnanomaterials as model systems for developing technologically useful nanomaterials.Scientific work on this subject can be traced back over 100 years.In 1861 theBritish chemist Thomas Graham coined the term colloid to describe a solutioncontaining 1 to 100 nm diameter particles in suspension. Around the turn of thecentury, such famous scientists as Rayleigh, Maxwell, and Einstein studied colloids.In 1930 the Langmuir-Blodgett method for developing monolayer films wasdeveloped. By 1960 Uyeda had used electron microscopy and diffraction to studyindividual particles. At about the same time, arc, plasma, and chemical flame furnaceswere employed to prouduce submicron particles. Magnetic alloy particles for use inmagnetic tapes were produced in 1970.By 1980, studies were made on clusterscontaining fewer than 100 atoms .In 1985, a team led by Smalley and Kroto foundC clusters were unusually stable. In 1991, Lijima spectroscopic evidence that 60reported studies of graphitic carbon tube filaments.Research on nanomaterials has been stimulated by their technologicalapplications. The first technological uses of these materials were as catalysts andpigments. The large surface area to volume ratio increases the chemicalactivity.Because of this increased activity, there are significant cost advantages infabricating catalysts from nanomaterials. The peoperties of some single-phasematerials can be improved by preparing them as nanostructures. For example, thesintering temperature can be decreased and the plasticity increased on single-phase,structural ceramics by reducing the grain size to several nanometers. Multiphasenanostructured materials have displayed novel behavior resulting from the small sizeof he individual phases.Technologically useful properties of nanomaterials are not limited to theirstructural, chemical, or mechanical behavior. Multilayers represent examples ofmaterials in which one can modify of tune a property for a specific application bysensitively controlling the individual layer thickness. It was discovered that the resistance of Fe-Cr multilayered thin films exhibited large changes in an applied magnetic field of several tens of kOe.This effect was given the name giant magnetoresistance (GMR). More recently, suitably annealed magnetic multilayers have been developed that exhibit significant magnetoresistance effects even in fields as low as 5 to10 Oe (Oersted). This effect may prove to be of great technological importance for use in magnetic recording read heads.In microelectronics, the need for faster switching times and ever larger integration has motivated considerable effort to reduce the size of electronic components. Increasing the component density increases the difficulty of satisfying cooling requirements and reduces the allowable amount of energy released on switching between states. It would be ideal if the switching occurred with the motion of a single electron. One kind of single-electron device is based on the change in the Coulombic energy when an electron is added or removed from a particle. For a nanoparticle this enery change can be large enough that adding a single electron will effectively blocks the flow of other electrons. The use of Coulombic repulsion in this way is called Coulomb blockade.In addition to technology, nanomaterials are also interesting systems for basic scientific investigations .For example, small particles display deviations from bulk solid behavior such as reductios in the melting temperature and changes (usually reductions) in the lattice parameter. The changes n the lattice parameter observed for metal and semiconductor particles result from the effect of the surface free energy. Both the surface stress and surface free energy are caused by the reduced coordination of the surface atoms. By studying the size dependence of the properties of particles, it is possible to find the critical length scales at which particles behave essentially as bulk matter. Generally, the physical properties of a nanoparticle approach bulk values for particles containing more than a few hundred atoms.New techniques have been developed recently that have permitted researchers to produce larger quantities of other nanomaterials and to better characterize these materials.Each fabrication technique has its own set of advantages anddisadvantages.Generally it is best to produce nanoparticles with a narrow size distribution. In this regard, free jet expansion techniques permit the study of very small clusters, all containing the same number of atoms. It has the disadvantage of only producing a limited quantity of material.Another approach involves the production of pellets of nanostructured materials by first nucleating and growing nanoparticles in a supersaturated vapor and then using a cold finger to collect the nanoparticle. The nanoparticles are then consolidated under vacuum. Chemical techniques are very versatile in that they can be applied to nearly all materials (ceramics, semiconductors, and metals) and can usually produce a large amount of material. A difficulty with chemical processing is the need to find the proper chemical reactions and processing conditions for each material. Mechanical attrition, which can also produce a large amount of material, often makes less pure material. One problem common to all of these techniques is that nanoparticles often form micron-sized agglomerates. If this occurs, the properties of the material may be determined by the size of the agglomerate and not the size of the individual nanoparticles. For example, the size of the agglomerates may determine the void size in the consolidated nanostructured material.The ability to characterize nanomaterials has been increased greatly by the invention of the scanning tunneling microscope (STM) and other proximal probes such as the atomic force microscope (AFM), the magnetic force microscope, and the optical near-field microscope.SMT has been used to carefully place atoms on surfaces to write bits using a small number of atmos. It has also been employed to construct a circular arrangement of metal atoms on an insulating surface. Since electrons are confined to the circular path of metal atoms, it serves ad a quantum ‘corral’of atoms. This quantum corral was employed to measure the local electronic density of states of these circular metallic arrangements. By doing this, researchers were able to verify the quantum mechanical description of electrons confined in this way.Other new instruments and improvements of existing instruments are increasingly becoming important tools for characterizing surfaces of films, biological materials, and nanomaterials.The development of nanoindentors and the improvedability to interpret results from nanoindentation measurements have increased our ability to study the mechanical properties of nanostructured materials. Improved high-resolution electron microscopes and modeling of the electron microscope images have improved our knowledges of the structure of the the particles and the interphase region between particles in consolidated nanomaterials.Nanotechnology1. IntroductionWhat id nanotechnology? it is a term that entered into the general vocabulary only in the late 1970’s,mainly to describe the metrology associated with the development of X-ray,optical and other very precise components.We defined nanotechnology as the technology where dimensions and tolerances in the range 0.1~100nm(from the size of the atom to the wavelength of light) play a critical role.This definition is too all-embracing to be of practical value because it could include,for example,topics as diverse as X-ray crystallography ,atomic physics and indeed the whole of chemistry.So the field covered by nanotechnology is later narrowed down to manipulation and machining within the defined dimensional range(from 0.1nm to 100nm) by technological means,as opposed to those used by the craftsman,and thus excludes,for example,traditional forms of glass polishing.The technology relating to fine powders also comes under the general heading of nanotechnology,but we exclude observational techniques such as microscopy and various forms of surface analysis.Nanotechnology is an ‘enabling’ technology, in that it provides the basis for other technological developments,and it is also a ‘horizontal’or ‘cross-sectional’technology in that one technological may,with slight variations,be applicable in widely differing fields. A good example of this is thin-film technology,which is fundamental to electronics and optics.A wide range of materials are employed in devices such as computer and home entertainment peripherals, including magnetic disc reading heads,video cassette recorder spindles, optical disc stampers and ink jet nozzles.Optical and semiconductor components include laser gyroscope mirrors,diffraction gratings,X-ray optics,quantum-well devices.2. Materials technologyThe wide scope of nanotechnology is demonstrated in the materials field,where materials provide a means to an end and are not an end in themseleves. For example, in electronics,inhomogeneities in materials,on a very fine scale, set a limit to the nanometre-sized features that play an important part in semiconductor technology, and in a very different field, the finer the grain size of an adhesive, the thinner will be the adhesive layer, and the higher will be the bond strength.(1) Advantages of ultra-fine powders. In general, the mechanical, thermal, electrical and magnetic properties of ceramics, sintered metals and composites are often enhanced by reducing the grain or fiber size in the starting materials. Other properties such as strength, the ductile-brittle transition, transparency, dielectric coefficient and permeability can be enhanced either by the direct influence of an ultra-fine microstructure or by the advantages gained by mixing and bonding ultra-fine powders.Oter important advantages of fine powders are that when they are used in the manufacture of ceramics and sintered metals, their green (i.e, unfired) density can be greatly increased. As a consequence, both the defects in the final produce and the shrinkage on firing are reduced, thus minimizing the need for subsequent processing.(2)Applications of ultra-fine powders.Important applications include:Thin films and coatings----the smaller the particle size, the thinner the coating can beElectronic ceramics ----reduction in grain size results in reduced dielectric thicknessStrength-bearing ceramics----strength increases with decreasing grain sizeCutting tools----smaller grain size results in a finer cutting edge, which can enhance the surface finishImpact resistance----finer microstructure increases the toughness of high-temperature steelsCements----finer grain size yields better homogeneity and densityGas sensors----finer grain size gives increased sensitivityAdhesives----finer grain size gives thinner adhesive layer and higher bond strength3. Precision machining and materials processingA considerable overlap is emerging in the manufacturing methods employed in very different areas such as mechanical engineering, optics and electronics. Precision machining encompasses not only the traditional techniques such as turning, grinding, lapping and polishing refined to the nanometre level of precision, but also the application of ‘particle’ beams, ions, electrons and X-rays. Ion beams are capable of machining virtually any material and the most frequent applications of electrons and X-rays are found in the machining or modification of resist materials for lithographic purposes. The interaction of the beams with the resist material induces structural changes such as polymerization that alter the solubility of the irradiated areas.(1) Techniques1) Diamond turning. The large optics diamond-turning machine at the Lawrence Livermore National Laboratory represents a pinnacle of achievement in the field of ultra-precision machine tool engineering. This is a vertical-spindle machine with a face plate 1.6 m in diameter and a maximum tool height of 0.5m. Despite these large dimensions, machining accuracy for form is 27.5nm RMS and a surface roughness of 3nm is achievable, but is dependent both on the specimen material and cutting tool.(2) GrindingFixed Abrasive Grinding The term“fixed abrasive” denotes that a grinding wheel is employed in which the abrasive particles, such as diamond, cubic boron nitride or silicon carbide, are attached to the wheel by embedding them in a resin or a metal. The forces generated in grinding are higher than in diamond turning and usually machine tools are tailored for one or the other process. Some Japanese work is in the vanguard of precision grinding, and surface finishes of 2nm (peak-to-valley) have been obtained on single-crystal quartz samples using extremely stiff grinding machinesLoose Abrasive Grinding The most familiar loose abrasive grinding processes are lapping and polishing where the workpiece, which is often a hard material such asglass, is rubbed against a softer material, the lap or polisher, with abrasive slurry between the two surfaces. In many cases, the polishing process occurs as a result of the combined effects of mechanical and chemical interaction between the workpiece, slurry and polished.Loose abrasive grinding techniques can under appropriate conditions produce unrivalled accuracy both in form and surface finish when the workpiece is flat or spherical. Surface figures to a few nm and surface finishes bettering than 0.5nm may be achieved. The abrasive is in slurry and is directed locally towards the workpiece by the action of a non-contacting polyurethane ball spinning at high speed, and which replac es the cutting tool in the machine. This technique has been named “elastic emission machining” and has been used to good effect in the manufacture of an X-ray mirror having a figure accuracy of 10nm and a surface roughness of 0.5nm RMS.3)Thin-film production. The production of thin solid films, particularly for coating optical components, provides a good example of traditional nanotechnology. There is a long history of coating by chemical methods, electro-deposition, diode sputtering and vacuum evaporation, while triode and magnetron sputtering and ion-beam deposition are more recent in their wide application.Because of their importance in the production of semiconductor devices, epitaxial growth techniques are worth a special mention. Epitaxy is the growth of a thin crystalline layer on a single-crystal substrate, where the atoms in the growing layer mimic the disposition of the atoms in the substrate.The two main classes of epitaxy that have ben reviewed by Stringfellow (1982) are liquid-phase and vapour-phase epitaxy. The latter class includes molecular-beam epitaxy (MBE), which in essence, is highly controlled evaporation in ultra high vacuum. MBE may be used to grow high quality layered structures of semiconductors with mono-layer precision, and it is possible to exercise independent control over both the semiconductor band gap, by controlling the composition, and also the doping level. Pattern growth is possible through masks and on areas defined by electron-beam writing.4. ApplicationsThere is an all-pervading trend to higher precision and miniaturization, and to illustrate this a few applications will be briefly referred to in the fields of mechanical engineering,optics and electronics. It should be noted however, that the distinction between mechanical engineering and optics is becoming blurred, now that machine tools such as precision grinding machines and diamond-turning lathes are being used to produce optical components, often by personnel with a backgroud in mechanical engineering rather than optics. By a similar token mechanical engineering is also beginning to encroach on electronics particularly in the preparation of semiconductor substrates.(1) Mechanical engineeringOne of the earliest applications of diamond turning was the machining of aluminum substrates for computer memory discs, and accuracies are continuously being enhanced in order to improve storage capacity: surface finishes of 3nm are now being achieved. In the related technologies of optical data storage and retrieval, the toler ances of the critical dimensions of the disc and reading head are about 0.25 μm. The tolerances of the component parts of the machine tools used in their manufacture, i.e.the slideways and bearings, fall well within the nanotechnology range.Some precision components falling in the manufacturing tolerance band of 5~50nm include gauge blocks, diamond indenter tips, microtome blades, Winchester disc reading heads and ultra precision XY tables (Taniguchi 1986). Examples of precision cylindrical components in two very different fields, and which are made to tolerances of about 100 nm, are bearing for mechanical gyroscopes and spindles for video cassette recorders.The theoretical concept that brittle materials may be machined in a ductile mode has been known for some time. If this concept can be applied in practice it would be of significant practical importance because it would enable materials such as ceramics, glasses and silicon to be machined with minimal sub-surface damage, and could eliminate or substantially reduce the need for lapping and polishing.Typically, the conditions for ductile-mode machining require that the depth of cutis about 100 nm and that the normal force should fall in the range of 0.1~0.01N. These machining conditons can be realized only with extremely precise and stiff machine tools, such as the one described by Yoshioka et al (1985), and with which quartz has been ground to a surface roughness of 2 nm peak-to-valley. The significance of this experimental result is that it points the way to the direct grinding of optical components to an optical finish. The principle can be extended to other materials of significant commercial importance, such as ceramic turbine blades, which at present must be subjected to tedious surface finishing procedures to remove the structure-weakening cracks produced by the conventional grinding process.(2) OpticsIn some areas in optics manufacture there is a clear distinction between the technological approach and the traditional craftsman’s approach, particul arly where precision machine tools are employed. On the other hand, in lapping and polishing, there is a large grey area where the two approaches overlap. The large demand for infrared optics from the 1970s onwards could not be met by the traditional suppliers, and provided a stimulus for the development and application of diamond-turning machines to optic manufacture. The technology has now progressed and the surface figure and finishes that can be obtained span a substantial proportion of the nanotechnology range. Important applications of diamond-turned optics are in the manufacture of unconventionally shaped optics, for example axicons and more generelly, aspherics and particularly off-axis components. Such as paraboloids.The mass production(several million per annum) of the miniature aspheric lenses used in compact disc players and the associated lens moulds provides a good example of the merging of optics and precision engineering. The form accuracy must be better than 0.2μm and the surface roughness m ust be below 20 nm to meet the criterion for diffraction limited performance.(3) ElectronicsIn semiconductors, nanotechnology has long been a feature in the development of layers parallel to the substrate and in the substrate surface itself, and the need for precision is steadily increasing with the advent of layered semiconductor structures.About one quarter of the entire semiconductor physics community is now engaged in studying aspects of these structures. Normal to the layer surface, the structure is produced by lithography, and for research purposes ar least, nanometre-sized features are now being developed using X-ray and electron and ion-beam techniques.5. A look into the futureWith a little imagination, it is not difficult to conjure up visions of future developments in high technology, in whatever direction one cares to look. The following two examples illustrate how advances may take place both by novel applications and refinements of old technologies and by development of new ones.(1) Molecular electronicsLithography and thin-film technology are the key technologies that have made possible the continuing and relentless reduction in the size of integrated circuits, to increase both packing density and operational speed. Miniaturization has been achieved by engineering downwards from the macro to the micro scale. By simple extrapolation it will take approximately two decades for electronic switches to be reduced to molecular dimensions. The impact of molecular biology and genetic engineering has thus provided a stimulus to attempt to engineer upwards, starting with the concept that single molecules, each acting as an electronic device in their own right, might be assembled using biotechnology, to form molecular electronic devices or even biochip computers.Advances in molecular electronics by downward engineering from the macro to the micro scale are taking place over a wide front. One fruitful approach is by way of the Langmure-Biodgett (LB) film using a method first described by Blodgett (1935).A multi-layer LB structure consists of a sequence of organic monolayers made by repeatedly dipping a substrate into a trough containing the monolayer floating on a liquid (usually water), one layer being added at a time. The classical film forming materials were the fatty acids such as stearic acid and their salts. The late 1950s saw the first widespread and commercially important application of LB films in the field of X-ray spectroscopy (e.g, Henke 1964, 1965). The important properties of the films that were exploited in this application were the uniform thickness of each film, i.e.one molecule thick, and the range of thickness, say from 5to 15nm, which were available by changing the composition of the film material. Stacks of fifty or more films were formed on plane of curved substrates to form two-dimensional diffraction gratings for measuring the characteristic X-ray wavelengths of the elements of low atomic number for analytical purposes in instruments such as the electron probe of X-ray micro-analyzer.(2) Scanning tunneling engineeringIt was stated that observational techniques such as microscopy do mot, at least for the purposes of this article, fall within the domain of nanotechnology. However,it is now becoming apparent that scanning tunneling microscopy(STM) may provide the basis of a new technology, which we shall call scanning tunneling engineering.In the STM, a sharp stylus is positioned within a nanometre of the surface of the sample under investigation. A small voltage applied between the sample and the stylus will cause a current to foow through the thin intervening insulating medium (e.g.air, vacum, oxide layer). This is the tunneling electron current which is exponentially dependent on the sample-tip gap. If the sample is scanned in a planr parallel to ies surface and if the tunneling current is kept cnstant by adjusting the height of the stylus to maintain a constant gap, then the displacement of the stylus provides an accurate representation of the surface topographyu of the sample. It is relevant to the applications that will be discussed that individual atoms are easily resolved by the STM, that the stylus tip may be as small as a single atom and that the tip can be positioned with sub-atomic dimensional accuracy with the aid of a piezoelectric transducer.The STM tip has demonstrated its ability to draw fine lines, which exhibit nanometre-sized struture, and hence may provide a new tool for nanometre lithography.The mode of action was not properly understood,but it was suspected that under the influence of the tip a conducting carbon line had been drawn as the result of polymerizing a hydrocarbon film, the process being assisted by the catalytic activity of the tungsten tip. By extrapolating their results the authors believed that it would be possible to deposit fine conducting lines on an insulating film. The tip would operatein a gaseous environment that contained the metal atoms in such a form that they could either be pre-adsorbed on the film or then be liberated from their ligands or they would form free radicals at the location of the tip and be transferred to the film by appropriate adjustment of the tip voltage.Feynman proposed that machine tools be used to make smaller machine tools which in turn would make still smaller ones, and so on all the way down to the atomic level. These machine tools would then operate via computer control in the nanometre domain, using high resolution electron microscopy for observation and control. STM technology has short-cricuired this rather cumbrous concept,but the potential applications and benefits remain.原文(二)Micro-machine1. IntroductionFrom the beginning, mankind seems instinctively to have desired large machines and small machines. That is, “large” and “small” in comp arison with human-scale. Machines larger than human are powerful allies in the battle against the fury of nature; smaller machines are loyal partners that do whatever they are told.If we compare the facility and technology of manufacturing larger machines, common sense tells us that the smaller machines are easier to make. Nevertheless, throughout the history of technology, larger machines have always stood ort. The size of the restored models of the water-mill invented by Vitruvius in the Roman Era, the windmill of the middle Ages, and the steam engine invented by Watt is overwhelming. On the other hand, smaller machined in history of technology are mostly tools. If smaller machines are easier to make, a variety of such machined should exist, but until modern times, no significant small machines existed except for guns and clocks.This fact may imply that smaller machines were actually more difficult to make. Of course, this does not mean simply that it was difficult to make a small machine; it means that it was difficult to invent a small machine that would be significant to human beings.。
材料科学与工程专业英语Unit2ClassificationofMaterials译文
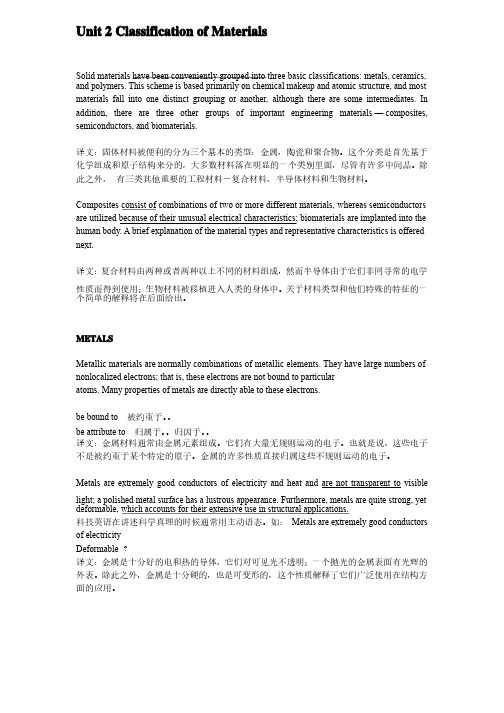
Unit 2 Classification of MaterialsSolid materials have been conveniently grouped into three basic classifications: metals, ceramics, and polymers. This scheme is based primarily on chemical makeup and atomic structure, and most materials fall into one distinct grouping or another, although there are some intermediates. In addition, there are three other groups of important engineering materials —composites, semiconductors, and biomaterials.译文:译文:固体材料被便利的分为三个基本的类型:金属,陶瓷和聚合物。
固体材料被便利的分为三个基本的类型:金属,陶瓷和聚合物。
固体材料被便利的分为三个基本的类型:金属,陶瓷和聚合物。
这个分类是首先基于这个分类是首先基于化学组成和原子结构来分的,化学组成和原子结构来分的,大多数材料落在明显的一个类别里面,大多数材料落在明显的一个类别里面,大多数材料落在明显的一个类别里面,尽管有许多中间品。
尽管有许多中间品。
除此之外,此之外, 有三类其他重要的工程材料-复合材料,半导体材料和生物材料。
有三类其他重要的工程材料-复合材料,半导体材料和生物材料。
Composites consist of combinations of two or more different materials, whereas semiconductors are utilized because of their unusual electrical characteristics; biomaterials are implanted into the human body. A brief explanation of the material types and representative characteristics is offered next.译文:复合材料由两种或者两种以上不同的材料组成,然而半导体由于它们非同寻常的电学性质而得到使用;生物材料被移植进入人类的身体中。
未来的上海旅行英语作文
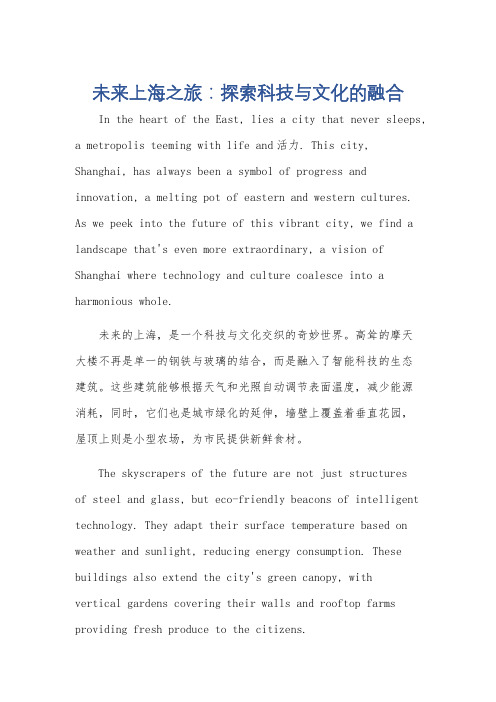
未来上海之旅:探索科技与文化的融合In the heart of the East, lies a city that never sleeps, a metropolis teeming with life and活力. This city, Shanghai, has always been a symbol of progress and innovation, a melting pot of eastern and western cultures. As we peek into the future of this vibrant city, we find a landscape that's even more extraordinary, a vision of Shanghai where technology and culture coalesce into a harmonious whole.未来的上海,是一个科技与文化交织的奇妙世界。
高耸的摩天大楼不再是单一的钢铁与玻璃的结合,而是融入了智能科技的生态建筑。
这些建筑能够根据天气和光照自动调节表面温度,减少能源消耗,同时,它们也是城市绿化的延伸,墙壁上覆盖着垂直花园,屋顶上则是小型农场,为市民提供新鲜食材。
The skyscrapers of the future are not just structuresof steel and glass, but eco-friendly beacons of intelligent technology. They adapt their surface temperature based on weather and sunlight, reducing energy consumption. These buildings also extend the city's green canopy, withvertical gardens covering their walls and rooftop farms providing fresh produce to the citizens.交通方面,未来的上海将是一个智能交通系统的典范。
J. Phys. Condens. Matter 15 (2003) R841–R896
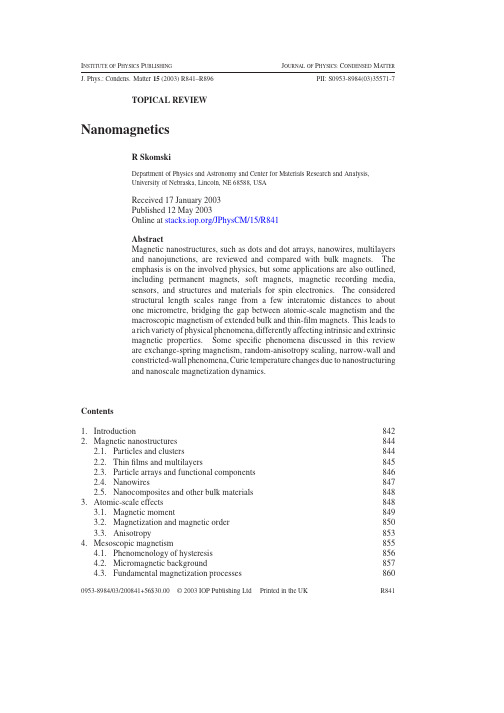
I NSTITUTE OF P HYSICS P UBLISHING J OURNAL OF P HYSICS:C ONDENSED M ATTER J.Phys.:Condens.Matter15(2003)R841–R896PII:S0953-8984(03)35571-7TOPICAL REVIEWNanomagneticsR SkomskiDepartment of Physics and Astronomy and Center for Materials Research and Analysis,University of Nebraska,Lincoln,NE68588,USAReceived17January2003Published12May2003Online at /JPhysCM/15/R841AbstractMagnetic nanostructures,such as dots and dot arrays,nanowires,multilayersand nanojunctions,are reviewed and compared with bulk magnets.Theemphasis is on the involved physics,but some applications are also outlined,including permanent magnets,soft magnets,magnetic recording media,sensors,and structures and materials for spin electronics.The consideredstructural length scales range from a few interatomic distances to aboutone micrometre,bridging the gap between atomic-scale magnetism and themacroscopic magnetism of extended bulk and thin-film magnets.This leads toa rich variety of physical phenomena,differently affecting intrinsic and extrinsicmagnetic properties.Some specific phenomena discussed in this revieware exchange-spring magnetism,random-anisotropy scaling,narrow-wall andconstricted-wall phenomena,Curie temperature changes due to nanostructuringand nanoscale magnetization dynamics.Contents1.Introduction8422.Magnetic nanostructures8442.1.Particles and clusters8442.2.Thinfilms and multilayers8452.3.Particle arrays and functional components8462.4.Nanowires8472.5.Nanocomposites and other bulk materials8483.Atomic-scale effects8483.1.Magnetic moment8493.2.Magnetization and magnetic order8503.3.Anisotropy8534.Mesoscopic magnetism8554.1.Phenomenology of hysteresis8564.2.Micromagnetic background8574.3.Fundamental magnetization processes860 0953-8984/03/200841+56$30.00©2003IOP Publishing Ltd Printed in the UK R841R842Topical Review4.4.Nucleation in nanocomposites and multilayers8634.5.Grain boundaries and nanojunctions8664.6.Textured magnets and random-anisotropy behaviour8694.7.Magnetic localization and cooperativity of magnetization reversal8715.Magnetization dynamics8745.1.Fundamental equations8755.2.Spin waves8765.3.Magnetic viscosity and sweep rate dependence of coercivity8785.4.Freezing behaviour8825.5.Conduction phenomena and spin electronics8836.Summary and conclusions884Acknowledgments886 Appendix.Magnetic materials886A.1.Permanent magnets886A.2.Magnetic recording media887A.3.Soft magnetic materials889References889 1.IntroductionThousands of years of human curiosity have led to the discovery of magnetism,and for many centuries magnetism has stimulated progress in science and technology.For a long time, the focus had been on macroscopic magnetism,as exemplified by the compass needle,by the geomagneticfield and by the ability of electromagnets and permanent magnets to do mechanical work.Atomic-scale magnetic phenomena,such as quantum-mechanical exchange[1–4], crystal–field interaction[5]and relativistic spin–orbit coupling[6,7],were discovered in thefirst half of the last century and are now exploited,for example,in advanced permanent–magnet intermetallics such as SmCo5and Nd2Fe14B[8].However,only in recent decades it became clear that solid-state magnetism is,to a large extent,a nanostructural phenomenon. The scientific and technological importance of magnetic nanostructures has three main reasons: (i)there is an overwhelming variety of structures with interesting physical properties,ranging from naturally occurring nanomagnets and comparatively easy-to-produce bulk nanocomposites to demanding artificial nanostructures,(ii)the involvement of nanoscale effects in the explanation and improvement of the properties of advanced magnetic materials,and(iii)nanomagnetism has opened the door for completely new technologies.A naturally occurring biomagnetic phenomenon is magnetite(Fe3O4)nanoparticles precipitated in bacteria,molluscs,insects and higher animals.Magnetostatic bacteria live in dark environments and contain chains of40–100nm magnetite particles used for vertical orientation[9].Similar magnetite particles have been found in the brains of bees,pigeons and tuna,and it is being investigated whether and how the particles serve asfield sensors for migration[10].Magnetite and other oxide particles are also responsible for rock magnetism, exploited for example in archaeomagnetic dating and for monitoring changes in the Earth’s magneticfield[11,12].Due to dilution and incomplete saturation,the thermoremanent magnetism of oxide particles in volcanic rocks is between0.0001and1µT,as compared to the geomagneticfield of the order of100µT.Typical particle sizes,varying between less than1and 100µm,are at the upper end of the structures of interest here,but the magnetization dynamics in these particles is a nanoscale phenomenon.Smaller oxide particle sizes,less than10nm,Topical Review R843Figure1.Two schematic bulk nanostructures:(a)sintered Sm–Co and(b)magnetic clusters(white)embedded in a matrix.The two structures are very different from the point of view ofsize,geometry,origin and functionality.The Sm–Co magnets,consisting of a rhombohedralSm2Co17-type main phase(grey),a Cu-rich SmCo5-type grain-boundary phase(black)and a Zr-rich hexagonal Sm2Co17-type platelet phase(white),are produced by a complicated annealingprocess and widely used in permanent magnets[8,26].Nanostructures such as that shown in(b)can be produced,for example,by mechanical alloying and are used as permanent magnets[27],soft magnets[24]and magnetoresistive materials[28,29].are observed in gels having the nominal composition FeO(OH)·n H2O[13].Fine particles are also encountered in superparamagnetic systems[14],ferrofluids[15]and meteorites[16].The further improvement of current magnetic materials heavily relies on nanostructuring. This refers not only to materials such as permanent magnets,soft magnets and recording media but also to emerging areas such as spin electronics.An example of improving the performance of magnetic materials by nanostructuring is hard–soft permanent-magnet composites[8,17–22].As analysed in[19],atomic-scale magnetism does not support substantial improvements of permanent magnets beyond existing intermetallics such as SmCo5,Sm2Co17and Nd2Fe14B, but adding a soft phase to a hard phase in a suitable nanostructure improves the permanent-magnet performance beyond that of the hard phase.This‘metamaterials’approach exemplifies the materials-by-design strategy and makes it possible to produce materials not encountered in nature.Other nanoscale effects are exploited in soft magnetic nanostructures,for example in Fe73.5Si13.5B9Cu1Nb3[23–25],where soft magnetic Fe3Si grains are embedded in an amorphous matrix.Figure1shows two structures of interest in this context.A fascinating approach is artificial nanostructuring to create completely new materials and technologies.One area is the ever-progressing miniaturization in computer technology,as epitomized by the use of nanostructured media for ultra-high density magnetic recording[30–39].A related area is spin electronics[40,41],and various types of nanostructures, such as multilayers and nanojunctions,are being investigated in this context.One problem of current interest is spin injection into nonferromagnetic materials[42,43] and magnetic semiconductors[44,45],whereas the use of spin degrees of freedom in quantum computing[46,47]remains a challenge to future research.Another area is magnetoresistive sensors exploiting magnetoresistance effects in metallic thinfilms[48–51],granular systems[28,29,52]and magnetic oxides[41,53–55].Some other present or future applications are nanoparticle ferrofluids for cancer treatment,guided by a magnet and delivering high local doses of drugs or radiation[56],micro-electromechanical systems(MEMS)and other nanodevices,and nanoscale magnetic-force nanotips made from PtCo[57,58].From a theoretical point of view,nanostructural phenomena are often described by differential equations of the type∇2φ−κ2φ=f(r),whereκ−1is an interaction length.ThisR844Topical Review must be contrasted to the inhomogeneous Laplace(or Poisson)equation∇2φ=f(r)which implies long-range interactions and describes,for example,electrostatic and magnetostatic phenomena.The interaction length reflects competition between different atomic energy contributions.When the competition is between the electrons’kinetic energy(hopping) and electrostatic energies(Coulomb interaction and exchange),thenκ−1scales as k F or a0. However,when the main competition involves relativistic interactions,then the interaction length increases to l0=a0/α,whereα=4πε0e2/¯h c≈1/137is Sommerfeld’sfine-structure constant[8,59].An example is the competition between magnetocrystalline anisotropy and exchange,because the spin–orbit coupling necessary to create anisotropy is a higher-order relativistic correction to the leading electrostatic contributions.Length scales of the order of l0=7.52nm are indeed encountered in many nanomagnetic problems[8,21,59],indicating that nanomagnetism goes beyond a‘mixture’of atomic and macroscopic physics.A related question concerns the transition from nanoscale to macroscopic behaviour.How many atoms are necessary to make a nanostructure indistinguishable from a bulk magnet?As we will analyse below,the answer to this reduced-dimensionality problem depends not only on the geometry of the structure but also on whether one considers intrinsic or extrinsic magnetic properties.Intrinsic properties,such as the spontaneous magnetization M s,thefirst uniaxial anisotropy constant K1and the exchange stiffness A,refer to the atomic origin of magnetism. As a rule,intrinsic properties are realized on length scales of at most a few interatomic distances and tend to approach their bulk values on a length scale of less than1nm,although there are exceptions to this rule[8,60].Extrinsic properties,such as the remanence M r and the coercivity H c,are nonequilibrium properties—related to magnetic hysteresis—and exhibit a pronounced real-structure dependence[8,61–63].For example,the coercivity of technical iron doubles by adding0.01wt%nitrogen[63].Such small concentrations have little effect on the intrinsic properties but lead to inhomogeneous lattice strains on a scale of many interatomic distances,affecting the propagation of magnetic domain walls and explaining the observed coercivity increase.Magnetic nanostructures exhibit a particularly rich extrinsic behaviour, including phenomena such as random-anisotropy scaling[64],remanence enhancement[17], micromagnetic localization[65],bulging-type nucleation modes[66]and a variety of grain-boundary[67]and exchange-coupling effects[68,69].This review deals with the physics of magnetic nanostructures.Section2is devoted to the geometrical aspect of nanomagnetism,introducing various types of nanostructures,section3 focuses on the relation between atomic physics and nanomagnetism and section4investigates nanoscale phenomena in a narrower sense.Section5discusses zero-andfinite-temperature dynamic effects,section6summarizes this work and draws some tentative conclusions.Finally, the appendix summarizes some information on materials of interest in nanomagnetism.2.Magnetic nanostructuresAdvanced magnetic nanostructures are characterized by a fascinating diversity of geometries, ranging from complex bulk structures(figure1)to a broad variety of low-dimensional systems. Figure2shows some examples.This section introduces typical geometries of interest in nanomagnetism and outlines their key features;the division into subsections is somewhat arbitrary,because many structuresfit into two or more categories.2.1.Particles and clustersVarious types of small magnetic particles exist in nature(section1)or are produced artificially. Fine-particle systems,such as Fe in Al2O3with particle diameters of the order of5nm, have been investigated over many decades[70].So-called‘elongated single-domain(ESD)Topical Review R845Figure2.Typical nanostructure geometries:(a)chain offine particles,(b)striped nanowire,(c)cylindrical nanowire,(d)nanojunction,(e)vicinal surface step,(f)nanodots,(g)antidots and(h)particulate medium.particles’[71]are used,for example,in magnetic recording.The properties of particles are also of interest for the investigation of nanowires(section2.4),dot arrays(section2.3)and thin-film(section2.2)and bulk(section2.5)composites.A crude criterion for the survival of the individuality of dots,particles and clusters in complex nanostructures is the strength of the exchange and magnetostatic interparticle interactions(section4.7).Interesting applications of small particles are stable colloidal suspensions known as ferrofluids[15,72].A variety of materials can be used,such as Fe3O4,BaFe12O19,Fe,Co and Ni,and a typical particle size is10nm.Most ferrofluids are based on hydrocarbons or other organic liquids,whereas water-based ferrofluids are more difficult to produce.They are used as liquids in bearings and to monitor magneticfields and domain configurations.Very small nanoparticles are also known as clusters.Their production by various techniques and typical structural properties have been reviewed by Sellmyer et al[39].In both free and embedded clusters,nanoparticle effects are particularly important.First,the large surface-to-volume ratio of clusters leads to a comparatively strong diameter dependence of the intrinsic properties such as anisotropy[73]and magnetization[74].Second,clusters tend to be superparamagnetic[14,75],particularly at high temperatures(section5.3).The ground-state domain configuration and the mechanism of magnetization reversal in small magnetic particles[75–80]depend on the particle size.At the macroscopic end of the range there are,e.g.,arrays of(110)Fe dots on sapphire,having a thickness of about 50nm and lateral dimensions of the order of1µm[78].Such dots are characterized byflux closure[78,81].In contrast,clusters are single-domain magnets(section4.2)and their reversal starts by coherent rotation(section4.3).2.2.Thinfilms and multilayersMany magnetic thinfilms and multilayers[51,82–87]can be considered as nanostructures, but since thin-film magnetism has developed into a separate branch of condensed matterR846Topical ReviewFigure3.An example of high resolution TEM from5.6nm Co clusters produced in our system.(Courtesy D J Sellmyer.)physics,a comprehensive introduction to these structures goes beyond the scope of this work. Nanostructured thinfilms with intermediate or high coercivities[20,21,88,89]have been studied in the context of permanent magnetism and magnetic recording.Thin-film structures exhibit a number of interesting properties.Examples are anisotropies of ideal and vicinal surfaces and of interfaces[84,90,91],moment modifications at surfaces and interfaces[92,93],thickness-dependentdomain-wall and coercive phenomena[59,82,86,94], interlayer exchange coupling[48–50]andfinite-temperature magnetic ordering[95].Two specific examples are the nanoscale exchange-coupling or‘exchange-spring’effects in multilayers[18,19,88,96–99]and the pinning of domain walls in sesquilayer iron–tungsten thinfilms[86].2.3.Particle arrays and functional componentsTwo-dimensional arrays of nanoparticles are interesting scientific model systems with many present or future applications.In particular,advanced magnetic recording media can be characterized as a complex array of magnetic particles,and interest in dot arrays[30,75,100–104]has been sparked by the search for ever-increasing storage densities in magnetic recording. In very small dots,quantum-mechanical effects are no longer negligible and there are phenomena such as quantum-well states.Quantum-dot effects are of interest in quantum computing and spin electronics[51,105].There are many methods for producing nanoparticle arrays[38,51,106].A traditional, though somewhat cumbersome,method to produce periodic arrays of nanoscale magnetic particles,dots and wires is nanolithography[107,108].Other examples are molecular-beam epitaxy[109],the use of STMs[110],chemical vapour deposition[101]and e-beam nanolithography[107,108].The call for well-characterized large-area arrays of nanoparticles has stimulated the search for advanced production methods such as laser-interference lithography(LIL),where laser-intensity maxima effect a local decomposition of a nonferromagnetic material into ferromagnetic islands[103].Another development is the use of ion beams[34,111],for example focused ion-beam(FIB)milling[111],to create small particles and particle arrays with well-defined properties.Most easily produced and investigated are submicron dots made from iron-series transition metals,such as Ni[101],but it is also possible to use intermetallics,such as permalloy[81,112], and to reduce the dot size to less than100nm.The arrays may be square or hexagonal,orTopical Review R847Figure4.Advanced MFM tips made by(a)ion milling,(b)electron beam deposition,(c)FIBmilling.(Courtesy S-H Liou.)the dots may form other structures such as corrals.Among the investigated phenomena are the properties of individual dots and interdot interactions[46,112,113].A related class of nanostructures is antidots,that is,holes in afilm rather than dots on afilm[109,114,115]. Antidots exhibit interesting resistive and magnetoresistive properties[114],but magnetic domains in antidots have been studied too[115].Potential applications include magnetic recording,sensors,magnetic and quantum computing,micron-and submicron-size mechanical devices,short-wavelength optics and spin electronics.In section4we will discuss some magnetic properties of dots and dot arrays.Other functional structures are,for example,nanojunctions[40,116],spin valves (section5.5)and tips for magnetic-force microscopy(MFM tips).Figure4shows three MFM tips made by various techniques[57].Some properties of nanojunctions and spin valves will be discussed in sections4.4and5.5.2.4.NanowiresThere is a smooth transition from elongated dots and thin-film patches[117,118]to nanowires[38,119–121].Magnetic nanowires are scientifically interesting and have potential applications in many areas of advanced nanotechnology,including patterned magnetic media, magnetic devices and materials for microwave applications.Thin-film nanowires,such as infigure2(b),are comparatively easily obtained by depositing magnetic materials on vicinal surfaces[51,117]and by exploiting structural anisotropies of the substrate[86].They can be produced with thicknesses down to one or two monolayers.Electrodeposition of magnetic materials into porous alumina may be used to produce regular wire arrays[38,119,121].Other ways of fabricating cylindrical nanowires include the deposition into molecular sieves[38,122–125],track-etched polymer membranes[126,127]and mica templates[128].By electrodeposition into porous anodic alumina[124,129,130]it is now possible to produce Fe,Co and Ni wires with diameters ranging from4to200nm,depending on the anodization conditions,and lengths of up to about1µm[38,106,119,121,131–135]. Typically,the nanowires form nearly hexagonal columnar arrays with variable centre-to-centre spacings of the order of50nm[38,121,131,135].The resulting materials are of interest as magnetic recording media[132,136],for optical and microwave applications[137,138]and as electroluminescent display devices[139].Aside from the above-mentioned iron-series transition-metal elements,there is interest in depositing alloys and multilayers,such as Fe/Pt, into porous templates[38,140,141].On the other hand,magnetoresistive effects have been investigated in electrodeposited Co–Cu alloy nanowires[142]and Co–Ni–Cu/Cu multilayered nanowires[143].R848Topical Review Much of the early work on magnetic nanowire arrays was concerned with exploratory issues,such as establishing an easy axis for typical preparation conditions,the essential involvement of shape anisotropy,as opposed to magnetocrystalline anisotropy,and the description of magnetostatic interactions between wires(see,e.g.,[38,127,135,144]and references therein).More recently,attention has shifted towards the understanding of magnetization processes[145–147].On a nanometre scale,interatomic exchange is no longer negligible compared to magnetostatic interactions.This leads to a transition from curling-type to quasi-coherent nucleation(section4.3).For Fe,Co and Ni,the corresponding diameters are about11,15and25nm,respectively,irrespective of the critical single-domain radius[8]. Furthermore,in section4.6we will see that the reversal behaviour is affected by the deposition-dependent polycrystallinity[38]of typical transition-metal nanowires[148].Some other interesting phenomena are magnetic-mode localization(section4.7),as evident,e.g.,from experimental activation volumes(section5.3),spin waves(section5.2)and current-induced magnetization reversal[149].2.5.Nanocomposites and other bulk materialsEmbedded clusters,granular materials and other bulk nanostructures are of great importance in nanoscience.The structural correlation lengths of typical nanocomposite materials range from about1nm in x-ray amorphous structures to several100nm in submicron structures and can be probed,for example,by small-angle neutron scattering(SANS)[150]and electron microscopy[21].Magnetic glasses[13,151]and atomic-scale defect structures are beyond the scope of nanomagnetics,but they are of indirect interest as limiting cases and because nanomagnetic phenomena have their quantum-mechanical origin in atomic-scale magnetism.Structures similar tofigure1(b)can be produced by methods such as mechanical alloying[152]and chemical reactions[27,153].Depending on grain size and microchemistry, they are used,for example,as permanent magnets(Nd–Fe–B),soft magnets(Fe–Cu–Nb–Si–B)and magnetoresistive materials(Co–Ag).There are two types of exchange-coupled permanent magnets:isotropic magnets[17,154–158],which exhibit random anisotropy and remanence enhancement(section4.6),and oriented hard–soft composites[19,21,88],which utilize exchange coupling of a soft phase with a high magnetization to a hard skeleton.Closely related systems with many potential applications are magnetic clusters deposited in a matrix. For example,the narrow size distribution of10–20%makes this material interesting as a granular media for magnetic recording[39].A well-known soft magnetic nanocomposite is the‘Yoshizawa’alloy Fe73.5Si13.5B9Cu1Nb3[23,159],which consists of iron–silicon grains embedded in an amorphous matrix.The Fe–Si nanocrystallites,which provide most of the magnetization,crystallize in the cubic DO3structure and have a composition close to Fe3Si.Nanoscale composites must be distinguished from amorphous metals(magnetic glasses) and spin glasses,whose exchange and anisotropy disorder is on an atomic scale[13,151,160–162].However,the boundary is smooth and spin glasses and amorphous materials exhibit various nanostructural phenomena.On the other hand,spin-glass-like phenomena are observed in some nanostructures.For example,interacting particles give rise to spin-glass-like(cluster-glass)dynamics[70,163]and isotropic nanostructures can be considered as random-anisotropy magnets[164].3.Atomic-scale effectsIntrinsic magnetic properties,such as magnetization and anisotropy,are determined on an atomic scale.For example,the magnetization ofα-Fe,µ0M s=2.15T,is associated with the body-centred cubic structure of elemental iron.However,some intrinsic effects are realizedTopical Review R849on a length scale of several interatomic distances.Examples are Ruderman–Kittel–Kasuya–Yosida(RKKY)interactions between localized moments embedded in a Pauli paramagnetic matrix and the disproportionally strong contribution of surface and interface atoms to the magnetic anisotropy of nanostructures.3.1.Magnetic momentThe magnetic moment m of solids nearly exclusively originates from the electrons in partly filled inner electron shells of transition-metal atoms.Of particular importance are the iron-series transition-metal or3d elements Fe,Co and Ni and the rare-earth or4f elements,such as Nd,Sm,Gd and Dy.Palladium series(4d),platinum series(5d)and actinide(5f)atoms have a magnetic moment in suitable crystalline environments.There are two sources of the atomic magnetic moment:currents associated with the orbital motion of the electrons and the electron spin.The magnetic moment of iron-series transition-metal atoms in metals(Fe,Co,Ni,YCo5) and nonmetals(Fe3O4,NiO)is largely given by the spin and the moment,measured inµB,is equal to the number of unpaired spins.For example,Fe2+(ferrous iron)has four unoccupied 3d↓orbitals,so that the moment per ion is4µB.The orbital moment is very small,typically of the order of0.1µB,because the orbital motion of the electrons is quenched by the crystal field[8,165,166].In contrast,rare-earth moments are given by Hund’s rules,which predict the spin and orbital moment as a function of the number of inner-shell electrons[165].The moment per atom is largely determined by intra-atomic exchange.Exchange is an electrostatic many-body effect,caused by1/|r−r |Coulomb interactions between electrons located at r and r .Physically,↓↑electron pairs in an atomic orbital are allowed by the Pauli principle but are unfavourable from the point of view of Coulomb repulsion.In the case of parallel spin alignment,↑↑,the two electrons are in different orbitals,which is electrostatically favourable,but the corresponding gain in Coulomb energy competes against an increase in one-electron energies.(Only one electron benefits from the low ground-state energy—the second electron must occupy an excited one-electron level.)The magnetic moments of insulating transition-metal oxides and rare-earth metals are located on well-defined atomic sites.However,in Fe,Co and Ni,as well as in many alloys,the moment is delocalized or itinerant.Itinerant ferromagnetism is characterized by non-integer moments and explained in terms of the metallic band structure[167–170].Nonmagnetic metals(Pauli paramagnets) have two equally populated↑and↓subbands;an applied magneticfield may transfer a few electrons from the↓band to the↑band,but the corresponding spin polarization is very small, of the order of0.1%.Itinerant ferromagnetism is realized by narrow bands,where the intra-atomic exchange is stronger than the bandwidth-related gain in single-electron hybridization (Stoner criterion).Atomic magnetic moments are affected by several nanoscale mechanisms.First, nonmagnetic atoms may become spin-polarized by neighbouring ferromagnetic atoms.A semiquantitative description of these effects is provided by the Landau–Ginzburg type[171] expression−A2∇2M+A0M=H ex(r).(3.1) Here M(r)is the induced magnetization(moment per unit volume),H ex is the intra-atomic exchangefield and A0and A2describe the electronic properties of the system.Essentially,χ(k,T)=1/(A0+k2A2)is the wavevector-dependent exchange-enhanced spin susceptibility, which is known for a variety of systems[172].Equation(3.1)predicts an exponential decay of the magnetization with a decay length of1/κ=(A2/A0)1/2.In simple metals,κscales as the Fermi wavevector(κ∼k F)and ferromagnetism is difficult to induce.However,exchange enhanced Pauli paramagnets,such as Pd and Pt,are very close to satisfying the Stoner criterion,R850Topical ReviewFigure5.Intrinsic properties of multilayered Pt–Fe structures(after[169]).so that A0andκare small[8,172].A similar A0reduction is encountered in semiconductors [44]and in semimetals such as Sb,where the decay length is of the order of1nm[173].Nanoscale moment modifications are important at surfaces and interfaces[173,174],but they do not extend very far into the bulk.By definition,Bloch wavefunctions extend to infinity, but nanoscalefinite-size effects yield only small corrections to the metallic moment.This can be seen,for example,from real-space approaches based on the moment’s theorem[8,175–178].When only nearest neighbours are taken into account,these methods yield the correct bandwidth but ignore details of the band structure,such as peaks in the density of states. Increasing the number of neighbours improves the resolution of the density of states and makes it possible to distinguish between bulk sites and sites close to surfaces.Figure5shows the modification of the moment and of the effective interatomic exchange in multilayered Fe–Pt magnets,as obtained fromfirst-principle electronic-structure calculations[169].As a rule,nanoscale intrinsic phenomena are caused by small differences between atomic interaction energies.In terms of(3.1),this occurs when A0≈0due to competing hopping and intra-atomic exchange energies.A loosely related phenomenon,observed for example in rare-earth elements and alloys,is noncollinear spin structures[13].Helimagnetic rare-earth noncollinearity is characterized by k vectors depending on the ratio of the nearest-and next-nearest-neighbour exchange(section3.2),and k may be,in principle,a very small fraction of k F.Even more complicated spin arrangements are possible in disordered magnets with competing interatomic exchange interactions(spin glasses)and at surfaces and interfaces. Furthermore,surface states[51,178]modify the magnetic moment of surface atoms[51,174]. Another type of noncollinearity is caused by spin–orbit coupling.The orbit of an electron, and therefore its crystal-field interaction,depend on the spin direction,so that electrons on sites without inversion symmetry can minimize the crystal-field energy by forming a slightly noncollinear spin structure.In spin glasses,this is known as Dzyaloshinskii–Moriya interaction[151],but the same effect occurs in other low-symmetry structures[179].Noncollinear states must not be confused with micromagnetic structures,such as domains and domain walls(section4.2).For example,small particles may exhibit some noncollinearity due to competing exchange,particularly at the surface,but an applied magneticfield merely changes the direction of the net magnetization,leaving the atomic-scale noncollinear correlations M(r i)·M(r j) unchanged.By contrast,micromagnetic magnetization processes,such as domain-wall motion,change the relative magnetization directions of well-separated spins in comparatively small magneticfields.3.2.Magnetization and magnetic orderIn a strict sense,ferromagnetism is limited to infinite magnets,because thermal excitations infinite magnets cause the net moment tofluctuate between opposite directions.In。
材料科学与工程专业英语第二版 翻译
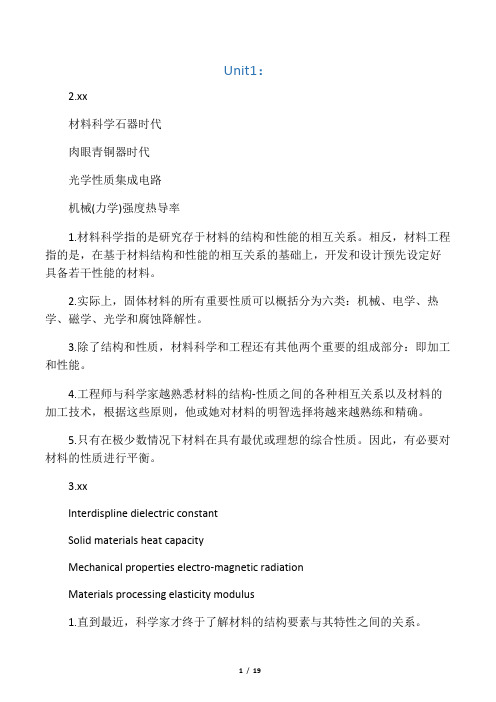
Unit1:2.xx材料科学石器时代肉眼青铜器时代光学性质集成电路机械(力学)强度热导率1.材料科学指的是研究存于材料的结构和性能的相互关系。
相反,材料工程指的是,在基于材料结构和性能的相互关系的基础上,开发和设计预先设定好具备若干性能的材料。
2.实际上,固体材料的所有重要性质可以概括分为六类:机械、电学、热学、磁学、光学和腐蚀降解性。
3.除了结构和性质,材料科学和工程还有其他两个重要的组成部分:即加工和性能。
4.工程师与科学家越熟悉材料的结构-性质之间的各种相互关系以及材料的加工技术,根据这些原则,他或她对材料的明智选择将越来越熟练和精确。
5.只有在极少数情况下材料在具有最优或理想的综合性质。
因此,有必要对材料的性质进行平衡。
3.xxInterdispline dielectric constantSolid materials heat capacityMechanical properties electro-magnetic radiationMaterials processing elasticity modulus1.直到最近,科学家才终于了解材料的结构要素与其特性之间的关系。
It was not until relatively recent times that scientists came to understand the relationship between the structural elements of materials and their properties .2.材料工程学主要解决材料的制造问题和材料的应用问题。
Material engineering mainly solve the problems of materials processing and materials application.3.材料的加工过程不但决定了材料的结构,同时决定了材料的特征和性能。
高温合金材料本构模型
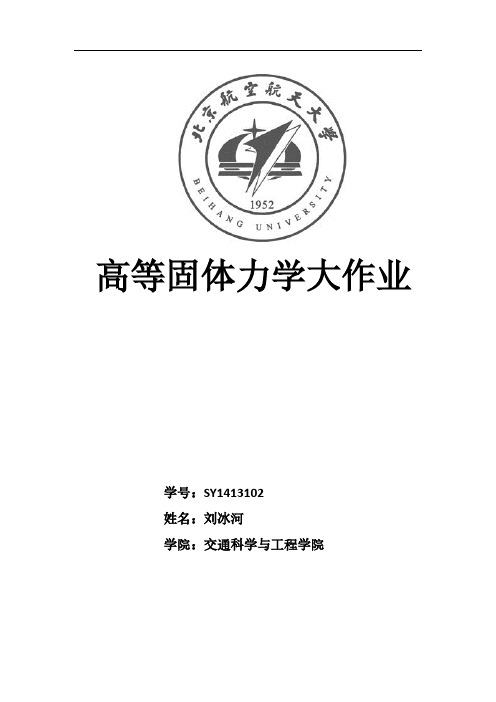
高等固体力学大作业学号:SY1413102姓名:刘冰河学院:交通科学与工程学院高温合金材料本构模型一.概述以上温度,高温合金于20世纪40年代问世,它指以铁、钴、镍为基体,能在600C一定应力条件下适应不同环境长时间或短时间使用的金属材料,具有较高的强度、塑性,良好的抗氧化、抗热腐蚀性能,良好的热疲劳性能,断裂韧性,良好的组织稳定性和使用可靠性。
其主要分为铁基高温合金、钴基高温合金和镍基高温合金[1, 2]。
本构关系广义上是指自然界作用与由该作用产生的效应两者之间的关系。
为确定物体在外部因素作用下的响应,除必须知道反映质量守恒、动量平衡、动量矩平衡、能量守恒等自然界普遍规律的基本方程外,还须知道描述构成物体的物质属性所特有的本构方程,才能在数学上得到封闭的方程组,并在一定的初始条件和边界条件下把问题解决。
因此,无论就物理或数学而言,刻画物质性质的本构关系是必不可少的。
目前应用较多的本构模型主要包括弹塑性力学中的经典本构模型,如理想弹塑性模型、线性强化弹塑性模型、幂强化力学模型和刚塑性力学模型。
然而塑性变形中应力-应变之间关系是非线性的,应变不仅与应力状态有关,而且和变形历史有关,因而研究者还提出增量理论和全量理论进行描述主要形成。
研究者在对金属材料的研究中不断提出新的本构模型,主要形成了两类本构模型:经验型本构模型,如Johnson -Cook 模型、Rusinek-Klepaczko 模型等;物理型的本构模型,如Hoge-Mukherjee模型、Zerilli-Armstrong 模型、MTS 模型等[3-5]。
二.几种本构模型介绍经典本构模型主要有理想弹塑性模型,线性强化模型,幂强化力学模型,以及刚塑性模型,如图一所示。
对于理想弹塑性模型来说, 在研究材料的应力应变关系时,分两个阶段。
第一阶段为弹性变形,第二阶段为塑性变形。
在塑性变形时,要考虑变形之前的弹性变形,而不考虑硬化的材料,也就是进入塑性状态后,应力不需要增加就可以产生塑性变形。
热处理-铁碳相图-Fe3C-奥氏体,马氏体,铁素体

Elongation
= 20 % in 2 in.;
Hardness
= Rockwell C 20, Rockwell B 95-100, or BHN 250-300.
Definition of structures
Austenite is an interstitial solid solution of
Cooling curve for pure iron
Definition of structures
Various phases that appear on the Iron-Carbon equilibrium phase diagram are as under: •Austenite •Ferrite •Pearlite •Cementite •Martensite* •Ledeburite
First horizontal line is at 1490°C, where peritectic reaction takes place: Liquid + d ↔ austenite
Second horizontal line is at 1130°C, where eutectic reaction takes place: liquid ↔ austenite + cementite
A map of the temperature at which different phase changes occur on very slow heating and cooling in relation to Carbon, is called Iron- Carbon Diagram.
Elongation
= 10 percent in 2 in.;
耐高温胶黏剂
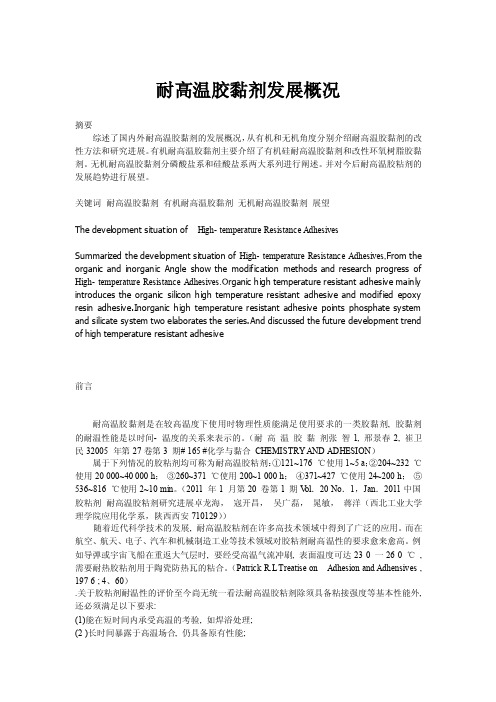
耐高温胶黏剂发展概况摘要综述了国内外耐高温胶黏剂的发展概况,从有机和无机角度分别介绍耐高温胶黏剂的改性方法和研究进展。
有机耐高温胶黏剂主要介绍了有机硅耐高温胶黏剂和改性环氧树脂胶黏剂。
无机耐高温胶黏剂分磷酸盐系和硅酸盐系两大系列进行阐述。
并对今后耐高温胶粘剂的发展趋势进行展望。
关键词耐高温胶黏剂有机耐高温胶黏剂无机耐高温胶黏剂展望The development situation of High- temperature Resistance AdhesivesSummarized the development situation of High- temperature Resistance Adhesives,From the organic and inorganic Angle show the modification methods and research progress of High- temperature Resistance anic high temperature resistant adhesive mainly introduces the organic silicon high temperature resistant adhesive and modified epoxy resin adhesive.Inorganic high temperature resistant adhesive points phosphate system and silicate system two elaborates the series.And discussed the future development trend of high temperature resistant adhesive前言耐高温胶黏剂是在较高温度下使用时物理性质能满足使用要求的一类胶黏剂, 胶黏剂的耐温性能是以时间- 温度的关系来表示的。
弱磁组英语

弱磁组英语The Weak Magnetic Group is a fascinating and diverse collection of elements that have unique properties and applications. These elements, which include vanadium, niobium, tantalum, and chromium, are known for their low magnetic susceptibility, making them valuable in various industries and technologies.Vanadium, the first member of the group, is a transition metal with a wide range of uses. It is a key component in the production of high-strength steel, where it enhances the metal's tensile strength, hardness, and resistance to corrosion. Vanadium-reinforced steel is widely used in the construction of bridges, buildings, and other infrastructure projects, ensuring the safety and longevity of these structures.In addition to its use in steel production, vanadium has found applications in the energy sector. Vanadium-redox flow batteries, a type of energy storage system, utilize the element's ability to exist in multiple oxidation states to store and release electrical energy efficiently. These batteries are particularly well-suited for large-scaleenergy storage, making them an attractive option for grid-scale applications and renewable energy integration.Niobium, the second member of the Weak Magnetic Group, is a refractory metal known for its high melting point and excellent corrosion resistance. These properties make it a valuable material in the aerospace industry, where it is used in the production of superalloys for jet engine components, rocket nozzles, and other high-temperature applications.Beyond its aerospace applications, niobium also plays a crucial role in the superconducting technology. Niobium-based superconducting materials are used in the construction of particle accelerators, magnetic resonance imaging (MRI) machines, and other scientific instruments that rely on the frictionless flow of electricity. The unique properties of niobium allow these devices to operate with unparalleled efficiency and precision, advancing our understanding of the physical world.Tantalum, the third member of the group, is a rare and valuable metal with a wide range of applications. Its high melting point, corrosion resistance, and biocompatibility make it a popular choice for use in various electronic devices, such as capacitors, integrated circuits, and medical implants. Tantalum-based capacitors are particularly notable for their ability to store large amounts ofelectrical charge in a compact form, making them essential components in modern consumer electronics and telecommunications equipment.In the medical field, tantalum has found use in the production of orthopedic implants, such as artificial hips and knees. The metal's biocompatibility and strength make it an ideal material for these applications, helping to improve the quality of life for patients in need of joint replacement surgery.Chromium, the final member of the Weak Magnetic Group, is a transition metal known for its hardness, corrosion resistance, and ability to form a wide range of compounds. One of the most significant applications of chromium is in the production of stainless steel, where it enhances the metal's resistance to oxidation and corrosion. Stainless steel, with its chromium content, is widely used in the construction of household appliances, kitchen equipment, and various industrial applications.Beyond its use in steel production, chromium also plays a crucial role in the manufacturing of pigments and coatings. Chromium-based pigments are used to impart vibrant colors to paints, inks, and ceramic glazes, contributing to the visual appeal of a wide range of products. Additionally, chromium-plated surfaces are often used in the automotive industry to provide a durable and attractive finish tovarious components.The Weak Magnetic Group's diverse range of applications highlights the importance of these elements in modern technology and industry. From the construction of high-strength steel to the development of advanced energy storage systems and medical implants, the elements in this group continue to play a vital role in shaping the world around us.As research and development in materials science continue to advance, it is likely that the Weak Magnetic Group will continue to be a focus of scientific exploration, leading to the discovery of new and innovative applications for these remarkable elements.。
碳酸锰的几种漂亮结构

case of large particle sizes. Although the nano-structured LiMn2O4 is very effective in improving the rate capability, the low tap density of nano-sized powders directly leads to the low energy density of a cell. To achieve a high tap density, electrode materials are preferred to have micron-sized particles, especially with spherical shapes which can pack more densely.21–25 In our study, we want to nd a balance between high tap density and short diffusion distance. Inspired by the work of Qian et al. who have synthesized hollow structures of Mn2O3, MnO2 and Mn2O3 microspheres,26–28 we design and synthesize a special yolk-structured LiMn2O4 microsphere e model, the outer shell is porous and composed of many nanoparticles, which can enlarge the specic surface area of the electrode and provide more reaction sites for lithium insertion and extraction. The core is relatively dense to increase the volumetric energy density. The spacing between the shell and the core can buffer any volume change of the core during heating/cooling or charge/discharge.
High-Temperature Superconductors
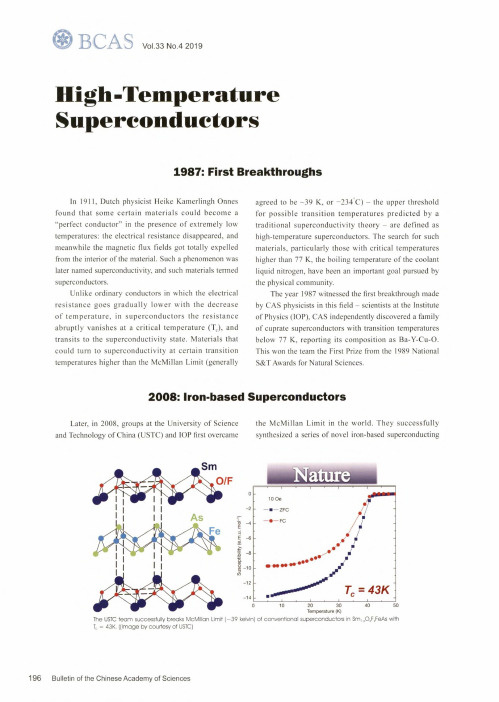
Vol.33 No.4 2019High-Temperature Superconduct ❶咔1987: First BreakthroughsIn 1911, Dutch physicist Heike Kamerlingh Onnesfound that some certain materials could become a "perfect conductor" in the presence of extremely lowtemperatures: the electrical resistance disappeared, andmean w hile the magnetic flux fields got totally expelled from the interior of the material. Such a phenomenon waslater named superconductivity, and such materials termedsuperconductors.Unlike ordinary conductors in which the electrical resistance goes gradually lower with the decreaseof temperature, in superconductors the resista nee abruptly vanishes at a critical temperature (T c ), and transits to the superconductivity state. Materials thatcould turn to superconductivity at certain transition temperatures higher than the McMillan Limit (generallyagreed to be ~39 K, or -234 C) - the upper threshold for possible transition temperatures predicted by atraditional superconductivity theory - are defined as high-temperature superconductors. The search for such materials, particularly those with critical temperatureshigher than 77 K, the boiling temperature of the coolant liquid nitrogen, have been an important goal pursued by the physical community.The year 1987 witnessed the first breakthrough madeby CAS physicists in this field 一 scientists at the Instituteof Physics (IOP), CAS independently discovered a familyof cuprate superconductors with transition temperatures below 77 K, reporting its composition as Ba-Y-Cu-O. This won the team the First Prize from the 1989 National S&T Awards for Natural Sciences.2008: Iron-based SuperconductorsLater, in 2008, groups at the University of Scienceand Technology of China (USTC) and IOP first overcamethe McMillan Limit in the world. They successfully synthesized a series of novel iron-based superconductingFs//sA eF -4-6-8L o lu.n .l u .®.Q =q w1020 3040 50Temperature (K)of conventional superconductors in Smj K O,F x FeAs withThe USTC team successfully breaks McMillan Limit (~39 kelvin) T c = 43K. ((Image by courtesy of USTC)196Bulletin of the Chinese Academy ofSciencesVol.33 No.4 2019 SPECIAL : CAS Ut 7010-3-8: Ca 10Pt 3As 8(Fe 2As 2) $ 10-4-8: Ca w Pt 4As 8(Fe 2As 2)5Atomic structures of selected major types of iron-based superc o n d ucting systems, many of which were discovered by the IOP/ USTC team. (Image by courtesy of IOP)Schematic structure of the Fe-As or Fe-Se layer in an iron-based superconductor, as revealed by IOP scientists. (Image by courtesy of IOP)materials, and identified superconductivity with a critical temperature (T c ) of 43 K (-230.15 C) in thesamarium oxygen fluorine iron arsenide (SmgOxFxFeAs) system; superconductivity with a critical temperatureof 41 K in CeFeAsO|.x F x ; and superconductivity withcritical temperatures above 50 K in two families of superconductors (namely REFeAsO|.x F x and REFeAsO* where RE represents rare earth elements). Particularly, the team once broke the world record of the highest criticaltemperature by successfully achieving superconductivity at a critical temperature as high as 55 K.With their explorations in superconductivity mechanisms, the team also verified and established iron-based superconductors as unconventional superconductors out of their basic physical characteristics.Due to their contributions to research in this field,the joint team won the First Prize from the 2013 NationalS&T Awards for Natural Sciences, filling a three-year long vacancy during which no recipient was deemed to beworthy of this prestigious prize.Bulletin of the Chinese Academy of Sciences197。
原位x射线相变表征技术

原位x射线相变表征技术## English Response.In situ X-ray phase characterization techniques offer a powerful tool for investigating the structural evolution of materials under various external stimuli, such as temperature, stress, electric fields, or magnetic fields.By monitoring the changes in the X-ray diffraction patterns, researchers can identify the formation and transformationof different crystal phases, determine the phase stability, and understand the underlying mechanisms of phase transitions. These techniques have found applications in a wide range of disciplines, including materials science, chemistry, physics, and engineering.One of the most common in situ X-ray phase characterization techniques is high-temperature X-ray diffraction (HTXRD). HTXRD allows for the investigation of phase transformations that occur at elevated temperatures. The sample is heated in a specialized furnace while the X-ray diffraction data is collected. By monitoring the changes in the diffraction patterns as a function of temperature, researchers can determine the temperature at which phase transitions occur, identify the crystal structures of the different phases, and study the kinetics of phase transformations.Another commonly used technique is in situ stress-XRD. In situ stress-XRD enables the characterization of phase transformations under mechanical stress. The sample is subjected to a controlled stress while the X-raydiffraction data is collected. By analyzing the changes in the diffraction patterns as a function of stress, researchers can determine the stress-induced phase transformations, identify the critical stress values for phase transitions, and understand the mechanisms of stress-induced plasticity and fracture.In situ electric field-XRD is a technique used to investigate phase transformations under the influence of electric fields. The sample is placed in a specialized cell that allows for the application of an electric field whilethe X-ray diffraction data is collected. By monitoring the changes in the diffraction patterns as a function of electric field strength, researchers can identify the electric field-induced phase transformations, determine the critical electric field values for phase transitions, and understand the mechanisms of electric field-induced polarization and switching.In situ magnetic field-XRD is a technique used to characterize phase transformations under the influence of magnetic fields. The sample is placed in a specialized cell that allows for the application of a magnetic field while the X-ray diffraction data is collected. By monitoring the changes in the diffraction patterns as a function of magnetic field strength, researchers can identify the magnetic field-induced phase transformations, determine the critical magnetic field values for phase transitions, and understand the mechanisms of magnetic field-induced magnetization and domain wall motion.In addition to the aforementioned techniques, there are a number of other in situ X-ray phase characterizationtechniques that have been developed for specific applications. These techniques include in situ X-ray microscopy, in situ X-ray scattering, and in situ X-ray absorption spectroscopy. Each of these techniques offers unique capabilities for investigating the structural evolution of materials under various external stimuli.The development of in situ X-ray phase characterization techniques has revolutionized the study of phase transformations and has provided valuable insights into the behavior of materials under a wide range of conditions. These techniques have enabled researchers to gain a deeper understanding of the fundamental mechanisms of phase transitions and have facilitated the development of new materials with tailored properties.## 中文回答:原位X射线相变表征技术为研究材料在各种外部刺激(如温度、应力、电场或磁场)下的结构演变提供了一种强大的工具。
建筑专业英语词汇(M-N)
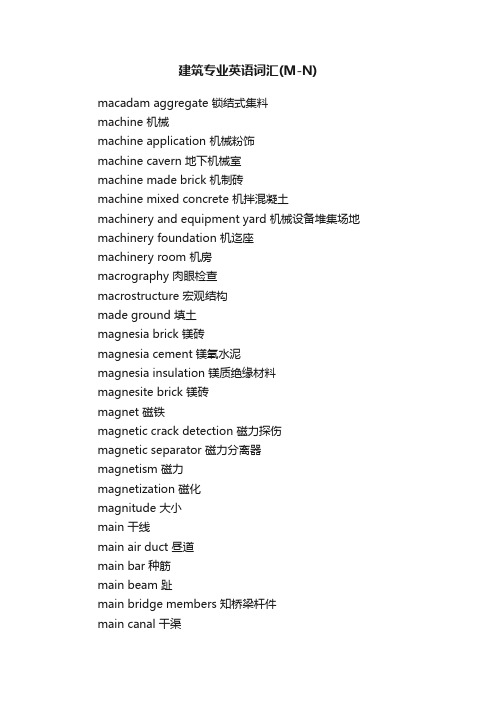
建筑专业英语词汇(M-N)macadam aggregate 锁结式集料machine 机械machine application 机械粉饰machine cavern 地下机械室machine made brick 机制砖machine mixed concrete 机拌混凝土machinery and equipment yard 机械设备堆集场地machinery foundation 机迄座machinery room 机房macrography 肉眼检查macrostructure 宏观结构made ground 填土magnesia brick 镁砖magnesia cement 镁氧水泥magnesia insulation 镁质绝缘材料magnesite brick 镁砖magnet 磁铁magnetic crack detection 磁力探伤magnetic separator 磁力分离器magnetism 磁力magnetization 磁化magnitude 大小main 干线main air duct 昼道main bar 种筋main beam 趾main bridge members 知桥梁杆件main canal 干渠main dike 痔main door 正门main drain 峙水沟main face 正面main girder 趾main pressure differential 周压差main rafter 知main reinforcement 种筋main runner 脂条main stair 芝梯main structural part 知结构配件main tie 值材main truss 朱架main valve 支main wall 纸mains distribution box 干线配电箱mains failure 电力网毁坏mains supply 干线供给mains water 自来水maintenance 维护maintenance cost 保养费maintenance hangar 修理棚maintenance overhaul 维修检查major axes of stress ellipse 应力椭圆的长径major axis 长径major overhaul 大修make up air 补偿空气make up pump 补充水泵make up water 补给水mall 木大锤mallet 木锤man 人man made stone 人造石management 管理manager program 操纵程序manhole 人孔manhole cover 检查井盖manhole rings 人孔环manhole step irons 人孔踏步铁manifold 集合管manilla rope 马尼拉麻绳索manometer 压力计manometric pressure 计示压力mansard 复折屋顶mansard dormer window 折线形屋顶窗mansard roof 折线形屋顶mansard roof construction 折线形屋顶结构mansion 住宅mantelpiece 壁炉台mantelshelf 壁炉台manual batcher 手动计量器manual cycling control 循环工程手动操纵manual damper 手动档板manual press 手压机manual proportioning control 手工称量控制manufacture 制造manufactured construction materials 人造建筑材料manufactured sand 人工砂manufacturing process 生产过程many roomed apartment 一套多房间公寓map 地图map cracking 网状裂缝marble 大理石marble gravel 大理石砾石marbling 仿制的大理石margin 边缘margin of safety 安全系数margin of stability 稳定性限marginal concrete strip finisher 混凝土边缘修整机marginal strip 路缘带marine clay 海成粘土marine construction 海洋建筑物marine glue 防水胶marine paint 海船油漆marine park 海蚀公园marine sand 海砂marine structures 海洋建筑物marine works 海洋建筑物mark 标记marker 标识器marker line 标志线marker post 标志杆market 市场market building 市场房屋marking 标识marking gauge 划线刀marl 泥灰岩marquee 雨罩marquetry 镶嵌装饰品marsh 沼泽地marshalling 砌体marshy ground 沼泽地mashroom valve 园锥形活门mason 石工mason's adjustable suspension scaffold 砖石工用可迭挂式脚手架mason's hammer 砖石工锤mason's mortar 圬工灰浆mason's scaffold 圬工脚手架masonry 砌石masonry anchor 圬工锚碇masonry arch 砌石拱masonry block 砌筑块masonry bridge 圬工桥masonry cement 砌切筑水泥masonry construction 砌筑结构masonry drill 圬工钻masonry mortar 圬工灰浆masonry nail 砖石钉masonry plate 座板masonry sand 圬工砂masonry veneer 表层砌体mass concrete 大块混凝土mass curve 土方曲线mass force 质量力mass foundation 块状基础mass haule curve 土方曲线mass produced structural units 成批生产的结构部件mass runoff 径量massive concrete structures 块状混凝土结构masstone 天然色调mast arm 灯具悬臂mast cap spider 紧桅箍星形轮mast with arm 带臂电杆master plan 总体规划mastic 玛脂mastic asphalt 石油沥青砂胶mastic cement 水泥砂胶mastic cooker 地沥青砂胶加热锅mat 底板mat coat 罩面mat foundation 板式基础mat reinforcement bender 钢筋网弯曲机match marking 装配标记match plane 开槽刨matchboarding 企口镶板matched ceiling 企口顶棚material 材料material aggressive to concrete 混凝土侵蚀材料material behavior 材料特性material debris chute 残渣滑槽material handling 材料装卸material handling bridge 材料转运桥material handling system 材料转运系统material hose 原料软管material list 材料酶表material retained on sieve 筛上筛余物material strength 材料强度material test 材料试验materials testing laboratory 材料试验室matt paint 无光泽涂料matte surface glass 毛面玻璃matted glass 毛面玻璃mattress 柴排mattress revetment 沉排铺面matured cement 长期贮存的老化水泥matured concrete 成熟混凝土maturing 老化maturing of concrete 混凝土的熟化maul 木大锤maximum 最大maximum allowable concentration 最大容许浓度maximum allowable emission 最大容许发散maximum capacity of well 井的最高容量maximum density grading 最大密度级配maximum density of soil 土壤的最大密度maximum dry density 最大干密度maximum flood discharge 最大洪水量maximum gradient 最大坡度maximum load 最大负荷maximum load design 最大荷载重设计法maximum rated load 最大额定荷载maximum safe load 最大安全载荷maximum simultaneous demand 最大同时需要量maximum size 最大尺寸maximum value 最大值meager lime 贫石灰mean cycle stress 循环应力的平均值mean depth 平均深度mean radiant temperature 平均辐射温度mean temperature difference 平均温差mean velocity of flow 平均临means of conveyance 传送工具means of egress 出口装置means of slinging 吊税置means of transportation 传送工具measure 尺寸measured drawing 实测图measurement 测定measurement data 测定资料measuring apparatus 测量仪器measuring chain 测量链measuring element 测定元件measuring frame 配料量斗measuring tank 计量槽measuring tape 卷尺measuring worm conveyor 测量螺旋输送机mechanical aeration 机械通风mechanical analysis 机械分析mechanical application 机械粉饰mechanical area 机械布置空间mechanical bond 机械结合mechanical calculation 力学计算mechanical classification 机械分级mechanical cooling 机械冷却mechanical core 技术设施中心带mechanical core wall 设有设施装置的心墙mechanical coupling link 机械轴节连杆mechanical draft cooling tower 机动气龄却塔mechanical dust collector 机械除尘器mechanical filter 机械滤器mechanical operator 机动装置mechanical rake 机械耙mechanical rammer 机械夯具mechanical refrigerating system 机械冷冻系统mechanical refrigeration 机械制冷mechanical stabilisation 机械稳定处理mechanical strength characteristics 力学强度特性mechanical testing 机械试验mechanical tooling of concrete 混凝土的机械加工mechanical treatment of sawage 污水的机械净化mechanical trowel 抹灰机mechanical ventilation 机械通风mechanics 力学mechanics of materials 材料力学mechanism 机构mechanization 机械化median barrier 路中护栏medium burned brick 标准烧成砖medium duty scaffold 中型脚手架medium grained asphalt concrete 中粒级配地沥青混凝土meeting rail 窗框的横挡meeting stile 连接板条member 构件membrane concrete curing 用膜混凝土养护membrane curing 薄膜养护法membrane filter 薄膜过滤器membrane fireproofing 隔膜防火层membrane forming type bond braker 粘结制止膜形成剂membrane theory 薄膜理论membrane waterproofing 薄膜防水memorial 纪念碑memorial architecture 纪念性建筑memorial table 纪念雕版menstruum 溶媒mercury arc lamp 汞弧灯meridian stress 经线应力mesh 筛目mesh analysis 筛分析mesh laying jumbo 钢筋网敷设设备mesh reinforced shotcrete 钢筋网加强喷射混凝土mesh reinforcement 钢筋网mesh series 筛一套mesh size 筛目大小metal 金属;铺路碎石metal clad cable 金属包皮电缆metal clad fire door 有金属包层的防火门metal construction 金属结构metal curtain wall 铁骨架悬挂壁metal door 金属门metal floor decking 金属楼板铺面metal form 金属模板metal furniture 金属家具metal gauze 金属网metal grating 金属栅板metal hose 金属蛇管metal lathing 钉钢丝网metal mesh fabric 金属网metal plate 金属板metal plating 镀metal runner 金属滑条metal sash putty 金属窗框油灰metal sheet 金属板metal sheet roof covering 金属薄板metal shingle 金属鱼鳞板metal stud 金属立杆metal tube 金属管metal valley 金属沟槽metal water stop 金属带密封metallic cement 熔渣硅酸盐水泥metallic sprayed coating 喷镀金属膜meteorological observation 气象观测meteorology 气象学meter 米methane tank 沼气桶method 方法method of curing 养护法method of elastic weights 弹性荷载法method of joint isolation 结点法method of least work 最小功法method of minimum strain energy 最小功法method of moment 力矩法method of redundant reactions 弯矩面积法method of sections 断面法method of the substitute redundant members 超静定构件取代法method of zero moment points 零点力矩法metro 地下铁道metropolitan area 大城市区域mezzanine 中二楼mica 云母micro crack 微细龟裂micro strainer 微孔滤网microclimate 小气候micrometer 微米microparticle 微粒micropolitan 居住区microscope 显微镜microstructure 微结构middle girder 中间梁middle strip 中间带middle surface 中面midget construction crane 小型建筑起重机midspan 中跨midspan deflection 中跨挠度midspan load 跨中荷载midwall 间隔墙mild steel reinforcement 低碳钢钢筋milk of lime 石灰乳mill 粉碎机mill construction 厂房结构millboard 麻丝板milled joint 研压接缝mineral aggregate 矿质骨料mineral dust 矿物粉末mineral fiber tiles 矿物纤维板mineral fibers 矿物纤维mineral filled asphalt 填充细矿料的沥青mineral filler 矿物质填料mineral pigment 矿物颜料mineral wool 矿物棉mineralogical composition of aggregates 集料的矿物的组成miniaturization 小型化mining shovel 采矿机铲mining town 矿业城镇mirror 镜mission tile 半圆形截面瓦mist 烟雾mist spraying 喷雾miter cut 斜切割miter gate 人字间门miter joint 斜角连接mitigation 水的软化mix 混合mix control 混合控制mix design 配合比设计mix in place machine 就地拌和机mix in place travel plant 就地拌和机mix ingredients 混合物成分mix proportions 混合比mixed cement 混合水泥mixed concrete 拌好的混凝土mixed construction 混合构造mixed greenery 混合式绿化区mixed in place construction of road 就地拌和法筑路mixed in place method 就地拌和法mixer 混合机mixer drum 混合圆筒mixer efficiency 混合机效率mixer skip 拌和机装料斗mixer trestle 混合机栈桥mixer truck 混凝土搅拌车mixing 混合mixing box 混合室mixing chamber 混合室mixing cycle 混合周期mixing damper 混合档板mixing drum 混合圆筒mixing of concrete 混凝土的搅拌mixing placing train 混合与灌筑综合装置mixing plant 搅拌装置mixing platform 拌和台mixing rate 混合物比率mixing ratio 混合比mixing screw 混合用螺旋mixing speed 拌和速度mixing tank 混合槽mixing temperature 混合温度mixing time 拌和时间mixing tower 混合塔mixing valve 混合阀mixing water 搅拌用水mixometer 拌和计时器mixture 混合mobile bituminous mixing plant 移动式沥青混合设备mobile crane 自行吊车mobile field office 移动式工地办公室mobile form 移动式模壳mobile hoist 移动式卷杨机mobile job crane 移动式起重机mobile load 活动荷载mobile scaffold tower 塔式机动脚手架mobile site office 移动式工地办公室mobile space heater 移动式空气加热器mobile tower crane 可移动塔式起重机mobile work platform 移动式工专mobility of concrete 混凝土怜性mode of buckling 压屈状态mode of failure 毁坏模式model 模型model testing 模型试验modern architecture 现代建筑modern city 现代城市modernization 现代化modification 更改modified i beam 改进的i型梁modified portland cement 改良硅酸盐水泥modular brick 符合模数尺寸的砖modular building 模数法建筑modular building unit 模数化建筑物单元modular constuction 模数化构造modular coordination 模数协调modular design 模数法设计modular dimension 模数尺寸modular ratio 模量化modular ratio method 模量比法modular size 标准化尺寸modular system 模数制modular unit 模数化构件modulator 爹器module 尺度modulus 系数modulus of creep 蠕变模量modulus of deformation 形变模量modulus of elasticity 弹性模量modulus of foundation support 地基反力系数modulus of resilience 回弹模量modulus of rigidity 抗剪模量modulus of rupture 挠折模量modulus of section 断面模量modulus of subgrade reaction 地基反力系数moist air cabinet 保湿箱moist room 湿气室moisture 湿气moisture barrier 防潮层moisture gradient 湿度梯度moisture laden air 潮湿空气moisture migration 水分移动moisture movement 水分移动moisture proof luminaire 防湿灯moisture resistant insulating material 耐湿性绝热材料moisture seal 防潮层moisture sensing probe 测湿器moisture tons 潜热冷冻负荷mold 模型mold oil 脱模油mold release 脱模剂molded brick 模制砖molded gutter 模制天沟molded insulation 模制塑料绝缘molded plywood 成型胶合板moldings 线脚molds reuseability 模重复使用能力mole 防波堤mole drain 地下排水沟mole drainage 地下排水工程moler brick 硅藻土砖moling 地下排水沟设置moment 力矩moment area 力矩面积moment area method 弯矩面积法moment at fixed end 支承挠矩moment at support 弯矩钢筋moment bar 弯矩杆moment buckling 力矩弯曲moment curvature law 力矩与曲率定律moment diagram 力矩分配法moment distribution 弯矩分配moment distribution method 力矩分配法moment equation 力矩方程moment influence line 力矩影响线图moment of couple 力偶矩moment of deflection 弯曲力矩moment of friction 摩擦力矩moment of inertia 惯性矩moment of load 负载矩moment of resistance 抗力矩moment of rupture 裂断力矩moment of span 跨矩moment of stiffness 刚性力矩moment reinforcement 弯曲钢筋moment resisting space frame 抗力矩空间构架moment resulting from sidesway 侧倾力矩moment splice 力矩的拼接moment zero point 零力矩点monastic building 寺院房屋monat cement 无熟料的矿渣水泥monitor 天窗monitor roof 通风的屋顶monitoring 监视monkey 起重机小车monkey wrench 螺丝板手monocable 单塑空死monolithic 整体式monolithic concrete 整体浇灌混凝土monolithic construction 整体式构造monolithic finish 整体修整monolithic grillage 整体式格床monolithic slab and foundation wall 整体式楼板与基础壁monolithic terrazzo 整块水磨石monolithic topping 整体式上部覆盖monopitch roof 单斜屋顶monorail 单轨道monorail system 单轨运输系统monorail transporter 单线运送机monorailway 单轨铁路monotower crane 单塔式起重机monsoon 季节风montage 装配monument 界标moon 月亮moor 沼泽地mooring accessories 系船设备mooring appurtenances 系船设备mooring dolphin 系船柱mooring ring 系船环mordant 腐蚀剂mortar 灰浆mortar additive 灰浆附加剂mortar admixture 灰浆附加剂mortar bar 灰浆棒mortar base 灰浆底座mortar bed 灰浆层mortar bond 灰浆砌筑mortar box 灰浆层mortar cube 灰浆立方块mortar fraction 灰浆部分mortar from chamotte 灰泥灰浆mortar from trass 火山灰灰浆mortar grouting 灌浆mortar gun 灰浆喷枪mortar mill 灰泥混合机mortar mixer 灰泥混合机mortar mixing machine 灰泥混合机mortar of dry consistency 干硬稠度灰浆mortar plasticizer 灰浆增塑剂mortar pump 灰浆泵mortar sand 灰浆用砂mortar spreader 灰浆撒布机mortar walling 灰浆砌筑mortarboard 灰板mortice 榫槽mortise 榫槽mortise chisel 錾mortise gauge 划榫器mortise joint 镶榫接头mortise lock 插锁mortise pin 梢子mosaic 镶嵌砖mosaic floor 镶嵌地板mosaic glass 嵌镶玻璃mosaic structure 镶嵌结构mosaic tile 镶嵌地砖moss 沼泽motel 汽车旅馆mother town 母域motion 运动motomixer 混凝土搅拌车motor 发动机motor bug 机动小车motor bus 公共汽车motor car 轿车motor damper 电动气邻器motor generator set 电动发电机motor grader 自行式平地机motor in head vibrator 电动振动棒motor operated valve 电动阀motor scraper 自动铲运机motorized solar control blinds 机动遮阳帘motorway 汽车路mottle effect 斑点样褪色mottled discoloration 斑点样褪色mottled surface 斑点样表面mould 型mould releasing agent 脱模剂moulded brick 模制砖moulded concrete 模制混凝土moulding plaster 模制用石膏moulding sand 型砂mound breakwater 堆石防波堤mounter 装配工mounting shop 装配车间mouthpiece 嘴子movable barrack 移动式工棚movable bridge 开合桥movable dam 活动堰movable distributor 活动配水器movable joint 活接头movable partition 可动间壁movable rocker bearing 活动振动机支承movable scaffolding 移动式脚手架movable span 可动桥跨movable tangential bearing 活动切线支承movable weir 活动堰moving form 移动式模壳moving form construction 活动模板建造moving load 活动荷载moving ramp 跳板moving staircase 自动升降梯moving walk 滑动步道muck loader 装渣机mucker 装渣机mucker belt 装岩机引带mucking 清理坍方mucosity 粘性mud 软泥mud box 泥渣分离箱mud outlet 污泥排泄口mud room 前厅mud soil 泥土mud trap 泥渣分离箱mudcapping 外部装药爆破mudsill 排架座木muffle furnace 高温烘炉muffler 消声器mullion 竖框mulseal 乳化沥青multi arch dam 连拱坝multi bucket dredger 多斗采砂船multi bucket excavator 多斗挖土机multi cell battery mould 多格仓式模板multi cell dust collector 多孔式积尘器multi compartment building 多户住宅multi compartment settling basin 多槽式沉淀池multi cored brick 多孔砖multi degree system 多自由度系multi lane roadway 多车道道路multi layer consolidation 多层固结multi legged sling 多支吊索multi point heater 多位置加热器multi purpose coating plant 多用途混合装置multi purpose scheme 综合利用设计multi rubber tire roller 多轴碾压机multi span structure 多跨结构multi wheel roller 多轴碾压机multi zone system 多区的空档统multiblade damper 多重叶瓣式节气闸multicolor brick 多色砖multicolor finish 多彩色终饰multideck screen 多层筛multielement member 多单元装配件multielement prestressing 多单元构件预加应力multifamily housing 多户住房multifolding door 卷折式门multilayer insulation 多层绝缘multilevel guyed tower 多位缆风拉紧的塔multimixer 通用混合器multipass aeration tank 多撂曝气池multiple 倍数multiple aeration tank 多撂曝气池multiple arch 连拱multiple arch bridge 连拱桥multiple bay frame 多跨框架multiple dome dam 弓顶连拱坝multiple dwelling 多户住房multiple dwelling building 多户住宅multiple flue chimney 多路烟囱multiple glass 多层玻璃multiple glazing 多层玻璃窗施工multiple leaf damper 多重叶瓣式节气闸multiple pipe inverted siphon 多管式倒虹吸管multiple purpose project 综合利用设计multiple purpose reservoir 综合利用水库multiple row heating coil 多行供热盘管multiple sedimentation tank 多路沉淀池multiple span bridge 多跨桥multiple span structure 多跨构造物multiple web systems 多肋式系统multiply plywood 多层夹板multiplying factor 倍加系数multistage construction 分阶段建筑multistage fan 多级风扇multistage gas supply system 多级供煤气系统multistage stressing 多级施加应力multistorey building 多层房屋;多层建筑物multistorey parking space 多层停车场multistoried building 多层房屋multistoried garage building 多层车库multiuse building 多用途建筑物multiway junction 复合交叉口municipal engineering 市政工程municipal facilities 市政工程设施municipal sanitary 城市卫生municipal sanitary engineering 城市卫生工程学municipal service 市政业务municipal sewage 城市污水municipal sewers 城市下水道网municipal treatment plant 城市污水处理场muntin 窗格条museum 博物馆mushroom construction 无粱板构造mushroom floor 环辐式楼板mushroom head column 无粱式楼板支承柱mushroom slab 无粱楼板mushy concrete 连混凝土mutual action of steel and concrete 钢筋和混凝土的共同酌n truss n字桁架n year flood n年周期洪水nail 钉nail claw 钉拔nail float 钉板抹子nail plate 钉接板nail puller 钉拔nailable concrete 受钉混凝土nailcrete 受钉混凝土nailed beam 钉梁nailed connection 钉结合nailed joint 钉结合nailed roof truss 钉固屋架nailer 可钉条nailing 打钉nailing block 可受钉块nailing marker 标记钉模naked flooring 毛面地板name block 名牌names of parts 部件品目narrow gauge 窄轨narrow gauge railway 窄轨铁路national architecture 民族建筑national highway 国道national style 民族形式natural adhesive 天然粘合剂natural aeration 自然通风natural aggregate 天然骨料natural angle of slope 自然倾斜角natural asphalt 天然沥青natural building sand 天然建筑砂natural cement 天然水泥natural circulation 自然循环natural color 天然色natural concrete aggregate 天然混凝土骨料natural convection 自然对流natural convector 自然对僚热器natural depth 天然深度natural draft 自然通风natural draft cooling tower 自然通风凉水塔natural drainage 自然排水natural finish 天然装饰natural flow 自然量natural foundation 天然地基natural grade 自然坡度natural ground 天然地基natural head 天然水头natural illumination factor 自然采光照玫数natural light 自然光natural lighting 天然照明natural mineral materials 天然矿物质材料natural pigment 天然颜料natural rock asphalt 沥青岩natural sand 天然砂natural sett 天然铺石natural slope 天然坡度natural stone 天然石natural stone slab 天然石板natural stone veneer 天然石表层natural stonework 天然石砌筑natural ventilation 自然通风natural water level 自然水位nature park 自然公园navier's hypothesis 纳维尔假说navigable canal 航行运河navigable construction 通航构造navigation canal 航行运河navigation facilities 通航设施navigation lock 通航船闸neat cement grout 净水泥浆neat cement mortar 净水泥灰浆neat cement paste 净水泥浆neat line 准线neat portland cement 纯波特兰水泥necking 颈缩现象needle 横撑杆needle beam 针梁needle beam scaffold 小梁托撑脚手架needle gate 针形闸门needle valve 针阀needle vibrator 针状振捣器needling 横撑杆negative friction 负摩擦negative moment 负力矩negative pressure 负压negative reaction 负反力negative reinforcement 负挠钢筋negative well 渗水井neighbourhood unit 近里单位neoprene paint 氯丁橡胶涂料nerve 交叉侧肋nervure 交叉侧肋nest of sieves 一套筛子net 网net area 净面积net cut 净挖方net duty of water 净用水量net fill 净填方net floor area 地板或楼层净面积net line 墙面交接线net load 净载荷net positive suction head 净吸引压头net residential area 居住净面积net room area 房间净面积net sectional area 净断面面积net settlement 净沉降net structure 网状结构netting 铁丝网network 格网络network of chains 三角测量网network of coordinates 坐标格网network of underground 地下管网neutral axis 中性轴neutral plane 中性面neutral pressure 中性压力neutral surface 中性面neutralisation tank 中和槽neutron shield 中子防护屏new construction 新建工程new town 新城市newel 螺旋楼梯中柱newel post 螺旋楼梯中柱newel stair 有中柱的螺旋楼梯newly placed concrete 新浇混凝土nibbed tile 有挂脚的瓦niche 壁龛nick break test 缺口冲辉验nidged ashlar 琢石nigged ashlar 琢石nigging 砍修石头night population 夜间人口night setback 夜间温度降低nippers 钳子nipple 短连接管nissen hut 金属结构掩蔽棚屋nitrogen 氮no bond prestressing 无粘结纲筋的后预应力no bond stretching 无粘结纲筋的后预应力no bond tensioning 无粘结纲筋的后预应力no fines concrete 无细集料混凝土no hinged frame 无铰的框架no slump concrete 干硬混凝土nodal forces 节点力node 节点nog 木栓nogging 填墙木砖;系柱横撑nogging piece 横撑noise 噪声noise abatement 减声noise abatement wall 减声壁noise absorption factor 噪声吸收系数noise attenuation 减声noise barrier 消音屏noise control 噪声控剂noise criteria curves 噪声标准曲线noise damper 消音器noise level 噪音级noise meter 噪声计noise nuisance 噪声危害noise rating 噪声评价noise rating curves 噪声评级曲线noise reduction coefficient 噪声降低系数nominal bore 公称直径nominal diameter 公称直径nominal dimension 标称尺寸nominal horsepower 标称马力nominal maximum size of aggregate 骨料的额定最大粒径nominal mix 标称配合比nominal size 公称尺寸nomogram 计算图表non air entrained concrete 非含气的混凝土non bonded joint 不粘着的楼缝non carbonate hardness 非碳酸盐硬度non clogging filter 不堵塞的过滤器non clogging screen 不堵塞的筛non concussive tap 无冲机头non fireproof construction 非防火建筑non load bearing partition 非承重隔墙non load bearing wall 非承重墙non pressure drain 无压排水渠non pressure pipe 无压管non redundant structure 静定结构non retern valve 逆止阀non scouring velocity 不冲刷临non shrinking cement 不收缩水泥non silting velocity 不淤临non slewing crane 非回转式吊机non slip floor 防滑楼板non structural component 非构造部件non tilting drum mixer 直卸料式拌合机nonagitating unit 途中不搅拌的混凝土运送车nonbearing partition 非承重隔墙nonbearing structure 非承压结构nonbearing wall 非承重墙nonbreakable glass 刚性玻璃noncohesive soil 不粘结的土质noncombustible construction 非燃结构noncombustible materials 非燃建筑材料noncreeping material 无蠕变材料nondestructive testing 非破坏性试验nondomestic building 非住宅楼nondomestic premises 非居住房产nonelastic deformation 非弹性变形nonelastic range 非弹性段nonhomogeneity of materials 材料的非均质性nonhomogeneous state of stress 不均等应力状况nonhydraulic lime 非水化石灰nonhydraulic mortar 非水硬性灰浆nonlinear distribution of stresses 应力的非线形分布nonlinear elastic behavior 非线性弹性状态nonlinear elasticity 非线性弹性nonlinear plastic theory 非线性塑性理论nonoverflow section 非溢水部分nonparallel chord truss 非平行弦杆桁架nonprestressed reinforcement 非预应力的钢筋nonresidential building 非居住建筑物nonrigid carriageway 非刚性车道nonrigid pavement 非刚性路面nonrotating rope 不旋转绳nonshrink concrete 抗缩混凝土nonsimultaneous prestressing 非同时预应力nonskid carpet 防滑毡层nonskid surfacing 防滑铺面nonstaining cement 无玷污水泥nonstaining mortar 不生锈的灰浆nonstandard component 非标准构件nonsticky soil 非粘结性土壤nonstorage calorifier 快速加热装置nonsymmetry 非对称nonvolatile matter 非挥发性物质nonvolatile vehicle 非挥发性媒液noon 正午noria 斗式提升机norm 标准norm of construction 建筑规范norm of material consumption 材料消耗定额norm of work 工专额normal concrete 普通混凝土normal consistency 正常稠度normal force 法向力normal force diagram 法向力图表normal heavy concrete 普通重混凝土normal portland cement 普通波特兰水泥normal section 正常断面normal weight concrete 正常重量混凝土normalization 标准化normalization of production 生产正规化normally hydrated cement 正常水化水泥north easter 东北风north light glazing 朝北锯齿形采光屋面装配玻璃north light gutter 朝北锯齿形屋顶边沟north wester 伪风northlight roof 朝北锯齿形屋面norton tube well 管井nose of groyne 丁顶前端noser 强迎面风nosing 梯级突边not water carriage toilet facility 非水冲厕notation 标记notch 切口notch board 梯级侧板notch fall 带缝隙的阶梯式构造notch impact strength 刻痕冲豢度notch shock test 刻痕冲辉验notch toughness 刻痕韧性notched bar test 切口试样冲辉验notched bars 变形钢筋notched specimen 切口试样notching 凹槽连结;刻痕notching machine 齿形刀片栽剪机novelty flooring 新颖花绞地板novelty siding 下垂坡叠板noxious fumes 有毒炮烟nozzle 喷嘴nozzle meter 管嘴式临计ntatorium 游泳池nuclear energy structures 原子力发电厂构造物nuclear reactor 核反应堆nursery 育儿室nursery garden 育苗区nut 螺母nut anchorage 带螺母锚nut runner 螺帽扳手nut socket driver 螺母扳手nut torque 拧螺母转矩nylon 尼龙nylon rope 尼龙绳。
纳米锰基普鲁士白的制备及电化学储钠性能

第34 7期2018年7月无机化学学报CHINESE JOURNAL OF INORGANIC CHEMISTRYVol.34 No.71327-1332纳米锰基普鲁士白的制备及电化学储钠性能陈新1徐丽1沈志龙2刘双宇1李慧1王博1谢健!,2姜银珠2刘海镇1盛鹏1赵广耀1全球能源互联网研究院有限公司,先进输电技术国家重点实验室,北京1022117(2浙江大学材料科学与工程学院,杭州3100277摘要:采用高温共沉淀法制备锰基菱方相的普鲁士白正极材料,研究合成温度对产物微结构和电化学性能的影响。
研究发现,随着合成温度的提高,产物的结晶度、颗粒尺寸和嵌钠容量明显提高。
当合成温度为90 "时,产物在M m A j-1下首次充放电容量分别达到142和U A m A lv i-1。
在30和'O m A.g-1分别循环300和600次时,容量仍保持在111和SAm Ah.g-1。
关键词:钠离子电池(正极材料(普鲁士白(电化学性能中图分类号:TB34 文献标识码:A 文章编号!1001-4861(2018)07-1327-06DOI:10.11862/CJIC.2018.177Preparation and Electrochemical Performance of theNanostructure Mn-Based Prussian WhiteCHEN Xin1XU Li1SHEN Zhi-Long2LIU Shuang-Yu1LI Hui1WANG Bo1XIE Jian!,2JIANG Yin-Zhu2LIU Hai-Zhen1SHENG Peng1ZHAO Guang-Yao1(^State Key Laboratory of A dvanced Transmission Technology, Global Energy InterconnectionResearch Institute Co. Ltd” Beijing 102211, China)(^School of M aterials Science and Engineering, Zhejiang University, Hangzhou310027, China) Abstract:Rhombohedral phase Mn-based Prussian white materials were synthesized by high-temperature coprecipitation method and the effect of synthesis temperature on the microstructure and electrochemical performance of the products was investigated. It is found that the crystallinity,particle size and Na-insertion capacity increase obviously with the increasing synthesis temperature. At a synthesis temperature of 90 ",the first charge and discharge capacities of the product reach 142 and 139 mAh'g-1at 15 mA'g-1.After 300 cycles at 30 mA'g-1 and 600 cycles at 50 mA'g-1,the discharge capacities are kept at 111 and 89 mAh'g-1,respectively. Keywords:sodium-ion batteries; cathode materials; Prussian white; electrochemical performance0引言随着能源和的日重,开发清洁、可持续能已成为球的研究[16。
材料专业英语--个人整理仅供参考
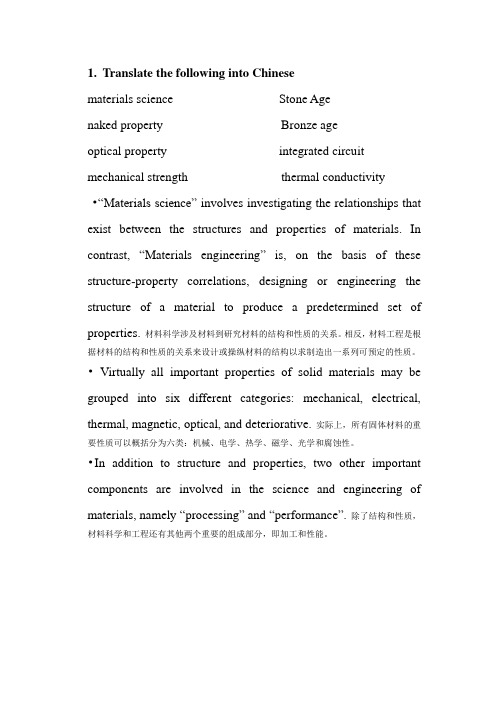
1.Translate the following into Chinesematerials science Stone Agenaked property Bronze ageoptical property integrated circuit mechanical strength thermal conductivity •“Materials science” involves investigating the relationships that exist between the structures and properties of materials. In contrast, “Materials engineering”is, on the basis of these structure-property correlations, designing or engineering the structure of a material to produce a predetermined set of properties.材料科学涉及材料到研究材料的结构和性质的关系。
相反,材料工程是根据材料的结构和性质的关系来设计或操纵材料的结构以求制造出一系列可预定的性质。
•Virtually all important properties of solid materials may be grouped into six different categories: mechanical, electrical, thermal, magnetic, optical, and deteriorative.实际上,所有固体材料的重要性质可以概括分为六类:机械、电学、热学、磁学、光学和腐蚀性。
•In addition to structure and properties, two other important components are involved in the science and engineering of materials, namely “processing” and “performance”.除了结构和性质,材料科学和工程还有其他两个重要的组成部分,即加工和性能。
英语作文磁铁怎么被利用
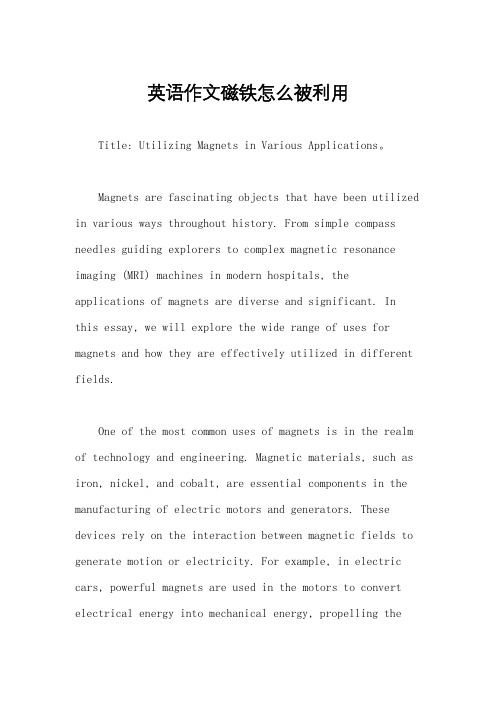
英语作文磁铁怎么被利用Title: Utilizing Magnets in Various Applications。
Magnets are fascinating objects that have been utilized in various ways throughout history. From simple compass needles guiding explorers to complex magnetic resonance imaging (MRI) machines in modern hospitals, theapplications of magnets are diverse and significant. Inthis essay, we will explore the wide range of uses for magnets and how they are effectively utilized in different fields.One of the most common uses of magnets is in the realm of technology and engineering. Magnetic materials, such as iron, nickel, and cobalt, are essential components in the manufacturing of electric motors and generators. These devices rely on the interaction between magnetic fields to generate motion or electricity. For example, in electric cars, powerful magnets are used in the motors to convert electrical energy into mechanical energy, propelling thevehicle forward efficiently.Moreover, magnets play a crucial role in data storage devices, such as hard disk drives (HDDs) and magnetic tape storage. Tiny magnets are used to represent binary data by aligning their magnetic fields either parallel or antiparallel to the surface of the storage medium. This enables the storage and retrieval of vast amounts of information in a compact and reliable manner.In the field of medicine, magnets are employed in various diagnostic and therapeutic applications. Magnetic resonance imaging (MRI) machines utilize powerful magnets to create detailed images of the internal structures of the body. By aligning the magnetic moments of hydrogen atoms in the body's tissues, MRI scanners can generate high-resolution images that aid in the diagnosis of medical conditions.Additionally, magnets are used in magnetic therapy, a form of alternative medicine that purportedly offers pain relief and promotes healing. Advocates of magnetic therapybelieve that applying magnets to specific areas of the body can improve blood circulation and alleviate discomfort. While the scientific evidence supporting the efficacy of magnetic therapy is still inconclusive, it remains apopular complementary treatment for certain ailments.Beyond technology and medicine, magnets findapplications in everyday objects and industries. Magnetic fasteners, such as those used in magnetic clasps forjewelry and magnetic closures for doors and cabinets, offer convenient and reliable solutions for securing items. Magnetic levitation (maglev) trains utilize powerful electromagnets to levitate and propel trains along tracks, reducing friction and increasing speed.Furthermore, magnets are essential in environmental and energy-related applications. Magnetic separation techniques are used in recycling plants to separate ferrous metalsfrom non-ferrous materials, allowing for efficient waste management and resource recovery. In renewable energy systems, such as wind turbines and hydroelectric generators, magnets are employed to convert kinetic energy intoelectrical energy, contributing to the production of clean and sustainable power.In conclusion, magnets are versatile tools with a wide range of applications across various fields. From technology and medicine to everyday objects and environmental endeavors, the utilization of magnets has become integral to modern society. As research and innovation continue to advance, it is likely that we will discover even more innovative ways to harness the power of magnets for the benefit of humanity.。
- 1、下载文档前请自行甄别文档内容的完整性,平台不提供额外的编辑、内容补充、找答案等附加服务。
- 2、"仅部分预览"的文档,不可在线预览部分如存在完整性等问题,可反馈申请退款(可完整预览的文档不适用该条件!)。
- 3、如文档侵犯您的权益,请联系客服反馈,我们会尽快为您处理(人工客服工作时间:9:00-18:30)。
Magnetic Structures of High Temperature Phases of TbBaCo2O5.5Minoru Soda1, Yukio Yasui1, Toshiaki Fujita1, Takeshi Miyashita1,Masatoshi Sato1 and Kazuhisa Kakurai21Department of Physics, Division of Material Science, Nagoya University,Furo-cho, Chikusa-ku, Nagoya 464-86022Advanced Science Research Center, Japan Atomic Energy Research Institute,Tokai-mura, Naka-gun, Ibaraki 319-1195AbstractNeutron diffraction studies have been carried out on a single crystal of oxygen-deficient perovskite TbBaCo2O5.5 in the temperature range of 7-370 K. There have been observed several magnetic or structural transitions. Among these, the existence of the transitions to the insulating phase from the metallic one at ~340 K, to the one with the ferromagnetic moment at ~280 K and possibly to the antiferromagnetic one at ~260 K, with decreasing temperature T correspond to those reported in former works. We have studied the magnetic structures at 270 K and 250 K and found that all Co3+ ions of the CoO6 octahedra are in the low spin state and those of the CoO5 pyramids carry spins which are possibly in the intermediate spin state. Non-collinear magnetic structures are proposed at these temperatures. Two other transitions have also been observed at the temperatures, ~100 K and ~250 K.corresponding author: M. Sato (e-mail: msato@b-lab.phys.nagoya-u.ac.jp)Keywords: TbBaCo2O5.5, neutron diffraction, magnetic structure, phase transitions1. IntroductionIn the perovskite oxides RCoO3 (R=Y and rare earth elements), the Co3+ ions have the low spin (LS; t2g6; S=0) ground state and often exhibit the spin state change1-6) to the intermediate spin (IS; t2g5e g1; S=1) state or the high spin (HS; t2g4e g2; S=2) state with increasing temperature T, indicating that the energy difference δE between the spin states is small. Because the energy splitting due to the crystal field generally increases with decreasing volume of the CoO6 octahedra, δE can be controlled by the volume change of the octahedra and therefore we can expect variety of physical behavior in the system related to the spin state change.The system R1-x A x CoO3-δ obtained by the substitution of R with divalent atoms A=Sr, Ba and Ca often exhibits the ferromagnetic and metallic properties for small δ with increasing doping concentration x due to the double exchange interaction.7-10) When Ba and relatively small trivalent elements are chosen as A and R, respectively, the system forms the oxygen-deficient perovskite structure, in which the oxygen deficiency is ordered.11-18) Among such oxides, RBaCo2O5(δ~0.5) (R=Y, Tb, Dy and Ho) exhibits transitions due to the antiferromagnetic ordering of the Co-moments and the ordering of Co2+ and Co3+.11-13) RBaCo2O5.5 (δ~0.25) (R=Y, Sm, Eu, Gd, Tb, Dy and Ho) also exhibits several transitions.14-18) However, only the restricted information is available on both the structural and the magnetic properties.In the present work, neutron scattering studies have been carried out on a single crystal of TbBaCo2O5.5 to collect basic information on the spin state of Co ions and the magnetic structures. The oxygen deficient perovskite structure of this system is formed by the linkage of alternating CoO6 octahedra and CoO5 pyramids along the b-axis, as shown schematically in Fig. 1.17,18) It has been reported that it has the orthorhombic unit cell, where the Ba and Tb are in different c-planes. The unit cell volume is described by ~a p×2a p×2a p, a p being the lattice parameter of the cubic perovskite cell. In this compound, the metal to insulator (MI) transition takes place with decreasing T at T MI~340 K,14,15) as shown in the inset of Fig. 2 by the resistivity(ρ) data taken for a sintered sample. The magnetizations M measured for a sintered sample and single crystal ones prepared in the present work are shown against T in the main panel of Fig. 2. As is described in detail later, the T-dependence of M indicates at least two magnetic transitions: The ferromagnetic transition occurs at T C~280 K, and the ferromagnetic phase suddenly changes to antiferromagnetic one at T N~260 K with decreasing T in zero magnetic field H as was confirmed by neutron diffraction study (T N seems to be slightly H-dependent.16)). Although transitions have been found at lower temperatures, too, for the crystals used in the present neutron diffraction studies, we mainly report here results of the studies on the magnetic structures at 270 K and 250 K, where the spin states of Co ions within the CoO6 octahedra and the CoO5 pyramids are basically clarified.2. ExperimentsA single crystal of TbBaCo2O5.5 was grown by a floating zone (FZ) method. Tb4O7, BaCO3 and Co3O4 were mixed with the proper molar ratios and the mixtures were pressed into rods and calcined at 1150°C for 10 h in flowing oxygen and cooled at a rate of 100 K/h. The single crystal was grown with use of an image furnace. The obtained crystal was annealed at 1000°C for 120 h in flowing oxygen and cooled at a rate of 100 K/h. The δ valueof the sample was determined by the thermo gravimetric analysis (TGA) to be δ~0.24±0.02 for the annealed one.The magnetizations M were measured by using a Quantum Design SQUID magnetometer in the temperature range of 5-350 K. The T-dependence of M taken at H=1 T and the H-dependence of M taken at several fixed T by using edge parts of the crystal after the zero field cooling are shown in Figs. 2 and 3, respectively. In taking the data of M, the crystal orientation with respect to the H-direction was so chosen that M at 270 K had the maximum value with the orientation-change within the condition of H⊥(c-axis). The T-dependence of Mof the as-cast crystal indicates that the ferromagnetic-antiferromagnetic transition at ~260 K is much broader than that of the polycrystal sample, while the transition of the annealed crystalis only slightly broader than that of the polycrystal sample. Then, we have used the annealed crystal in the neutron scattering studies. It is noted here that the anisotropy of the measured magnetization indicates that the ferromagnetic magnetization is within the c-plane.Neutron measurements were carried out by using the triple axis spectrometer TAS-2 installed at the thermal guide of JRR-3M of JAERI in Tokai. The crystal was oriented with the [100] (or [010]) axis vertical, where both (0,k,l) and (h,0,l) points in the reciprocal space could be observed due to the coexistence of the a*- and b*-domains. Several 0kl reflections can be distinguished from h0l reflections by the difference between the lattice parameters a and b/2 (The parameter a is slightly smaller than b/2.). The 002 reflection of Pyrolytic graphite (PG) was used for both the monochromator and the analyzer. The horizontal collimations usually used were 17’(effective)-40’-80’ (2-axis) and the neutron wavelength was ~2.44Å. A PG filter was placed in front of the sample to eliminate the higher order contamination. To realize the better resolution, horizontal collimations 17’(effective)-20’-20’-40’ were also used in some cases. Another PG filter was placed after the sample in measuring reflections for which the contamination of the higher order beam may not be negligible. The sample was set in an Al-can filled with exchange He gas, which was attached to the cold head of the Displex type refrigerator for the measurements below room temperature. The can was heated in a furnace for the measurements above room temperature.The volume ratio of the a*- and b*-domains was estimated at room temperature from theratios of the intensities of the 0k0 reflections with odd k values to those for 0k0/h00 with even k and corresponding h values. (In the measurements of the intensities, the crystal was oriented with the [001] axis vertical.)3. Experimental Results and DiscussionThe intensities of the neutron Bragg scattering have been measured on the single crystal of TbBaCo2O5.5 at various Q-points in the reciprocal space in the temperature range of 7-370 K, where several superlattice reflections as well as the fundamental reflections of the cell with the size of ~a p×2a p×2a p have been found. The points at which the reflections were observed at 7 K in the reciprocal space are shown in Fig. 4(a). (Q=(0,k,l) may be (k/2,0,l).) The temperature dependences of the peak intensities of the typical fundamental and superlattice reflections are shown in Figs. 4(b) and 4(c), respectively. With decreasing T from the temperature above 350 K, the intensities of several nuclear Bragg reflections change at T MI~340 K, and at T C~280 K, the additional reflection component appears at several nuclear Bragg points. It is considered to be magnetic, as is confirmed later by detailed analyses and corresponds to the appearance of the ferromagnetic moment. With further decreasing T, at T N~260 K, the additional component suddenly disappears and the superlattice peaks corresponding to the period of 2c (~4a p) along the c-axis appear (Q=(0,k,1/2), (0,k,3/2), etc.). At T~100 K, the superlattice peaks corresponding to the period of 4c along the c-axis appear (Q=(0,0,1±1/4), (0,0,3±1/4), (0,2,1±1/4), etc.) with decreasing T. The former group of the superlattice reflections may be magnetic, while we cannot distinguish if the latter superlattice reflections are magnetic or not. It should be also noted that the superlattice reflections corresponding to the modulation vector q= a*/3 or2b*/3 (the period of ~3a p in the real space) or q=a*/6 or b*/3 (the period of ~6a p in the real space) are observed even at temperatures higher than T MI. (We could not distinguish along which direction of a* or b* the modulation exists.) We have examined the possibility that these superlattice reflections of the ~3a p (or ~6a p) period originate from the coexistence of the tetragonal unit cell with the size of ~3a p×3a p×2a p reported in ref. 15 as the distinct phase with δ~0.28. However, by the detailed inspection of the X-ray diffraction patterns taken for the powder sample obtained by crushing a part of the crystal can exclude the possibility, because the volume of the tetragonal part is too small, if any, to explain the observed intensities of the superlattice reflections. These reflections of the ~3a p (or ~6a p) period are considered to be due to the existence of the modulation of the crystal structure of the main phase in the present crystal. However, we have not clarified detailed pattern of the modulation in the present study.The data of Fig. 4(b) suggest the existence of structural transitions in the present system at three temperatures, T MI, ~250 K and ~100 K. The transition at T MI has been reported by Kusuya et al.17) If the increase of the intensity of 020 reflection observed with decreasing Tbelow ~250 K, is due to the increase of the magnetic reflection, the system should have the net moment, because (0,2,0) corresponds to the ferromagnetic Bragg point in the reciprocal space. However, it is in contradiction to the present observation (see Fig.2). Then, the transition can be considered to be due to the structural one.As for the crystal structure above T MI~340 K, there exist several reports, 17,18) where the unit cell size is described by ~a p×2a p×2a p. Of course, the existence of the ~3a p (or ~6a p) period modulation along a- or b-axis observed in the present neutron diffraction measurements on the single crystal, cannot be explained. At this moment, we do not distinguish if the modulation is just a characteristic of the present single crystal sample or it exists in sintered samples, too. (Single crystals prepared from the molten phase may have different characteristics of the structure from those of sintered samples, because lattice imperfections such as the oxygen vacancies and inhomogeneity may be introduced in the course of the crystal growth.)For the precise analyses of the magnetic structures of the present system, its crystal structure has to be first determined. It is, however, not easy due to the presence of the ~3a p (or ~6a p) period modulation. Here, we simply neglect the modulation by considering that the intensities of the corresponding superlattice reflections are much weaker than the fundamental ones (less than ~ 0.5 % of the strongest one) and the crystal structure is analyzed by using the structure with the unit cell size of ~a p×2a p×2a p (space group P mmm17)). The results of the fitting of the calculated intensities (I cal) of the nuclear Bragg reflections to the integrated intensities (I obs) measured at 300 K are shown in Fig. 5, where I obs values are plotted against I cal. (In the fitting, we have considered the volume ratio of the a*- and b*-domains determined as stated in 2. The consideration of this domain distribution was also made in all the analyses described below.) The lattice parameters a, b and c are 3.8679±0.0012 Å, 7.8174±0.0025 Å and 7.5165±0.0016 Å, respectively, which should be compared with the values of 3.86769±0.00006 Å, 7.8143±0.0001 Å and 7.5125±0.0001 Å reported for the powder sample of ref. 17. The positional parameters obtained by the fitting are basically consistent with those in the article, though the fitting may not be fully satisfactory.Then, the magnetic structures have been analyzed based on the crystal structure obtained above at 270 K and 250 K. (Although the increase of the peak intensity shown in Fig. 4(b) at the (0,2,0) point in the reciprocal space indicates the occurrence of the structural transition to the new phase at ~250 K with decreasing T, no effect is expected on the analyses of the magnetic structure at 250 K, because the order parameter of the new phase is, at least, negligibly small at the temperature.) The integrated intensities of the magnetic scattering were obtained by taking the differences between the integrated intensities at 300 K and those at 270 K or 250 K. (This automatically indicates that the superlattice reflections corresponding to the ~3a p (or ~6a p) period modulation are not magnetic at least above 250 K.) The absorption- andLorentz factor-correction were made. The magnetic structures have been determined by assuming that the magnitudes of the Co-moments and the absolute values of their cant angle are equal at the crystallographically equivalent positions. For the magnetic form factor of Co3+, we used the isotropic values reported in ref. 19.At 270 K, in order to distinguish which reflection, 0kl and h0l, is actually observed, accurate determination of the scattering angles of the magnetic reflections has been carried out by using the finer horizontal collimations. The results found around (0,1,1) or (1/2,0,1) and (0,2,0) or (1,0,0) points in the reciprocal space are shown in the top and bottom panels of Fig. 6, respectively. In the figure, the solid circles represent the intensity profiles of the nuclear reflections observed at 300 K and the open circles are the intensity profiles of the magnetic scattering at 270 K, which are extracted by subtracting the neutron count number at 300 K from that observed at 270 K at each scattering angle 2θ. It can be concluded from the figures that the 1/201 and 020 magnetic reflections have significant intensities, while the intensities of the 011 and 100 reflections are zero or negligibly small (note that a<b/2). Then, because the ferromagnetic magnetization is known to be in the c-plane from the result shown in Fig. 2, the direction of the ferromagnetic moment has to be along the a-axis. Magnetic reflections have also been observed at (0,1,0) and (0,3,0) points but not observed at (1/2,0,0) and (3/2,0,0). Based on these results, the magnetic structure finally obtained here is shown in Fig. 7. Figure 8 shows the integrated intensities of the magnetic reflections (I obs) collected at 270 K against those of the model calculation (I cal) at several reflection points. The results are also shown in Table I. The fitting is as good as that for the nuclear structure shown in Fig. 5, indicating that the obtained magnetic structure is essentially correct, even though we have ignored the ~3a p (or ~6a p)modulation and just used the isotropic form factor of Co3+. The result also indicates that the transition is purely magnetic or at least mainly magnetic. One of the characteristics of this magnetic structure is that all Co3+ ions of CoO6 octahedra are in the LS state and the magnetic moments of Co3+ of CoO5 pyramids have the coplanar but canted structure. The magnitude µ of the aligned moments of Co3+ ions of the pyramids is 0.710(±0.019) µB. We think that Co3+ is in the IS state, even though the ordered moment is much smaller than the value in the fully ordered state. The absolute value of the canting angle φ1defined as shown in the bottom panel of Fig. 7 is 43.1(±2.0)°. The sign of φ1alternates along the a- and c-directions. The maximum value of the spontaneous magnetization in the c-plane, is expected to be 0.18 µB/Co by the present fitting. (Due to the existence of the domain structure, the magnetization has the maximum value when H is applied nearly along the [120] direction.) This result is consistent with the observed data shown in Fig. 3.The magnetic structure obtained at 250 K (or in the antiferromagnetic phase) is shown in Fig. 9, where all b-planes formed of the CoO5 pyramids have the same magnetic structure. Each c-plane has nonzero net magnetization and its direction rotates by 90°, when thez-position is shifted by ~c/2, as shown by the arrows in the right part of the figure. Figure 10 shows the integrated intensities of the magnetic reflections collected at 250 K (I obs) against those of the model calculation (I cal) at several superlattice points. (No magnetic reflections appear at the fundamental Bragg points.) The results are also shown in Table II. The fitting is as good as that for the nuclear structure shown in Fig. 5, indicating that the obtained magnetic structure is essentially correct. The result also indicates that the transition is purely magnetic or at least mainly magnetic. The Co3+ ions within the octahedra are in the LS state as 270 K and the magnitude of the aligned Co-moments within the pyramids is 1.090(±0.031) µB. The Co-moments cant by the angle φ2 = 33.5(±1.5)° from the a- or b-axis. Then, each CoO2 layer has the canted ferromagnetic structure similar to the structure of 270 K, in which the angle between the neighboring Co-moments along the a-axis is (180º-2φ2).We have so far discussed the magnetic structure of TbBaCo2O5.5 at 270 K (in the ferromagnetic phase) and 250 K (just below the ferromagnetic-antiferromagnetic transition temperature) by analyzing the neutron diffraction data taken for a single crystal sample. Studies on the magnetic or crystal structures below ~100 K and in the T-region between 100 K and 250 K, which have been found to be distinct from those of other phases in the present single crystal sample remain as the future problems.4. ConclusionThe neutron diffraction intensities have been measured on the single crystal of TbBaCo2O5.5 and five transitions have been found. They are the metal to insulator transition (structural transition) at 340 K, to the ferromagnetic phase at 280 K, to the antiferromagnetic one at 260 K, and two unidentified transitions at ~250 K and ~100 K , with decreasing T. The magnetic structures have been extracted at T=270 K (ferromagnetic phase) and T=250 K (antiferromagnetic one). We have found that the Co3+ ions of the CoO6 octahedra are in the LS state, and those of the CoO5 pyramids are possibly in the IS state at both temperatures. At T=270 K, the Co3+-moments of the CoO5 pyramids have the canted structure, where the direction of the ferromagnetic moment is along the a-axis. At T=250 K, they have the non-collinear structure, in which the direction of the net moment of the CoO2 layer rotates by 90° when the z-position increases by ~c/2.Acknowledgments - Work at the JRR-3M was performed within the frame of JAERI Collaborative Research Program on Neutron Scattering. We would like to thank Dr. M. Matsuda and Mr. Y. Shimojo for the technical assistance at the spectrometer TAS-2.References1) R. R. Heikes, R. C. Miller and R. Mazelsky: Physica 30 (1964) 1600.2) K. Asai, A. Yoneda, O. Yokokura, J. M. Tranquada, G. Shirane and K. Kohn: J. Phys. Soc.Jpn. 66 (1998) 290.3) M. Itoh, M. Sugahara, I. Natori and K. Morita: J. Phys. Soc. Jpn. 64 (1995) 3967.4) W. H. Madhusudan, K. Jagannathan, P. Ganguly and C. N. R. Rao: J. Chem. Soc. DaltonTrans. (1980) 1397.5) D. S. Rajoria, V. G. Bhide, G. R. Rao and C. N. R. Rao: J. Chem. Soc. Faraday Trans.70 (1974) 512.6) G. Thornton, F. C. Morrison, S. Partington, B. C. Tofield and D. E. Williams: J. Phys. C:Solid State Phys. 21 (1988) 2871.7) M. A. Señarís-Rodríguez and J. B. Goodenough: J. Solid State Chem. 118 (1995) 323.8) M. Itoh and I. Natori: J. Phys. Soc. Jpn. 64 (1995) 970.9) K. Yoshii, H. Abe and A. Nakamura: Mater. Res. Bull. 36 (2001) 1447.10) C. N. R. Rao, OM Parkash, D. Bahadur, P. Ganguly and S. Nagabhushana: J. Solid StateChem. 22 (1977) 353.11) E. Suard, F. Fauth, V. Caignaert, I. Mirebeau and G. Baidinozzi: Phys. Rev. B 61 (2000)R11871.12) T. V ogt, P. M. Woodwark, P. Karen, B. A. Hunter, P. Henning and A. R. Moodenbaugh:Phys. Rev. Lett. 84 (2000) 2969.13) F. Fauth, E. Suard, V. Caignaert, B. Domengès, I. Mirebeau and L. Keller: Eur. Phys. J. B21 (2001) 163.14) A. Maignan, C. Martin, D. Pelloquin, N. Nguyen and B. Raveau: J. Solid State Chem. 142(1999) 247.15) D. Akahoshi and Y. Ueda: J. Solid State Chem. 156 (2001) 355.16) M. Respaud, C. Frontera, J. L. García-Muñoz, M. Á. G. Aranda, B. Raquet, J. M. Broto, H.Rakoto, M. Goiran, A. Llobet and J. Rodríguez-Carvajal: Phys. Rev. B 64 (2001) 214401.17) H. Kusuya, A. Machida, Y. Moritomo, K. Kato, E. Nishibori, M. Takata, M. Sakata and A.Nakamura: J. Phys. Soc. Jpn. 70 (2001) 3577.18) C. Frontera, J. L. García-Muñoz, A. Llobet and M. A. G. Aranda: Phys. Rev. B 65 (2002)180405.19) P. J. Brown: International Tables for Crystallography, ed. by A. J. C. Wilson (Kluwer,Dordrecht, 1992) vol. C, chap. 4.Figure captionsFig.1 Schematic structure of TbBaCo2O5.5.Fig. 2 Temperature dependence of the magnetizations taken for a sintered sample and as-cast and annealed crystals (H=1 T). Inset shows the temperature dependence ofthe electrical resistivity measured for a sintered sample.Fig. 3 Magnetization of a single crystal of TbBaCo2O5.5 is shown at various temperatures against the magnetic field applied in the c-plane. In taking the data, the crystalorientation with respect to the H-direction was so chosen that M at 270 K has themaximum value with the orientation-change within the condition of H⊥(c-axis) Fig. 4 (a) Fundamental or superlattice points of the unit cell with the size of ~a p×2a p×2a p in the reciprocal space at 7 K. Solid circles represent the fundamental Bragg points.Other symbols are the superlattice points, where different symbols indicate thedifferent temperature dependence of the intensities. (b) and (c) The peak intensitiesof several reflections are shown against T.Fig. 5 Integrated intensities of the nuclear Bragg reflections collected at 300 K (I obs) are plotted against those (I cal) obtained by the fitting with the space group P mmm.Fig. 6 Profiles of the 011 or 1/201 (top) and the 020 or 100/020 (bottom) reflections. Solid circles represent the reflection intensities at 300 K and the open circles show themagnetic scattering intensities at 270 K, which are extracted by subtracting theneutron count number at 300 K from that observed at 270 K at each scattering angle2θ.Fig. 7 Schematic magnetic structure of TbBaCo2O5.5 at 270 K(in the ferromagnetic phase).Top: The direction of the Co3+-moments of the CoO5 pyramids in the b-plane.Bottom: The magnetic structure of a CoO2 layer.Fig. 8 Integrated intensities of the magnetic reflections collected at 270 K (I obs) are shown against those of the model calculation (I cal).Fig. 9 Schematic magnetic structure of TbBaCo2O5.5 at 250 K(in the antiferromagnetic phase). This structure has the period of ~4a p along the c-axis. The open arrowsdrawn in the right side of the figure show the directions of the net moments of theCoO2 layers. All Co3+ ions of CoO6 octahedra are in the low spin state.Fig. 10 Integrated intensities of the magnetic reflections collected at 250 K (I obs) are plotted against those of the model calculation (I cal).Table I Comparison of the observed intensities of the magnetic scattering with those calculated for the spin structure shown in Fig. 7.T=270 Kh k l I obs I cal*0 0 1 0± 26 70 0 2 340±457 7490 1 0 / 1/2 0 0 823± 57 7860 1 1 / 1/2 0 1 605± 49 6140 1 2 / 1/2 0 2 248± 43 2780 1 3 / 1/2 0 3 402±325 1800 1 4 / 1/2 0 4 138± 63 880 2 0 / 1 0 0 401± 77 3390 2 1 / 1 0 1 238±736 10 2 2 / 1 0 2 897±1720 3230 2 3 / 1 0 3 149±589 80 3 0 / 3/2 0 0 174± 28 1780 3 2 / 3/2 0 2 126± 39 1220 4 1 / 2 0 1 85± 86 <0.50 5 0 / 5/2 0 0 103± 58 59*for µ=0.710µB, φ1=43.1deg.Table II Comparison of the observed intensities of the magnetic scattering with those calculated for the spin structure shown in Fig. 9.T=250 Kh k l I obs I cal*0 0 1/2 2733±343 25150 0 3/2 561±79 7590 0 5/2 349±70 3760 0 7/2 225±74 2060 0 9/2 148±60 1200 1 1/2 / 1/2 0 1/2 1275±179 11410 1 3/2 / 1/2 0 3/2 755±78 8910 1 5/2 / 1/2 0 5/2 679±77 5400 1 7/2 / 1/2 0 7/2 258±70 3190 2 1/2 / 1 0 1/2 355±69 2710 2 3/2 / 1 0 3/2 272±64 2600 2 5/2 / 1 0 5/2 190±61 1990 3 1/2 / 3/2 0 1/2 255±56 247*for µ=1.090µB, φ2=33.5deg.c ba。