numerical simulation of hydrogen release in a private garage
中文翻译

非均质碳酸盐岩石润湿性分析的复杂性的研究Mohammadhossein Mohammadlou,挪威科技大学,SPE会员Mai Britt E. Mørk, 挪威科技大学,SPE会员版权归石油工程师学会所有,2012本文准备在4—7月份哥本哈根首都挪威举办的EAGE年会上发表并在欧洲石油工程师学会展出。
本文由SPE程序委员会选择发表,以下内容包含作者总结摘要。
文章内容未经石油工程师学会审核,由作者修正。
文章不涉及石油工程师学会管理人员或成员。
未经石油工程师学会书面同意,禁止电子传播、发布或保存文章任何内容。
打印摘要限制在300字以内,图表不允许复制,文章摘要必须明确标识SPE版权。
摘要近年来油藏润湿性评价及其对流体流动、烃类开采和流体分布的影响成为许多研究人员的研究主题,并且仍是油藏描述的一个主要的挑战。
本文通过比较特殊岩心分析(SCAL)和岩心栓电阻率指数测量,结合核磁共振(NMR)测井研究以及借助模块化动态工具(MDT)油藏测量获得的地层压力资料,研究非均质卡斯特化的碳酸盐岩的油藏润湿性。
SCAL研究结果表明油藏取心井段为亲水性油藏条件,电阻率指数测量研究结果也大致符合SCAL结果。
由于缺乏油藏主要部分的岩心数据,采用NMR T2分布的分析和MDT数据相结合来描述油藏的润湿性。
压力数据表明,除有关区域高电阻率和含油饱和度异常高压值以外,油藏剖面水柱压力梯度正常。
在油气聚集之后,已注水(油藏水位升高)区域,不应出现高含油饱和度。
这些井段的T2分布研究有助于标识油藏大孔隙的油湿的性质。
当孔隙表面为油湿时,油的表面弛豫会造成T2分布朝更短的T2sC转变。
在油湿孔隙,水容量弛豫延迟,相比水湿情况有更长的T2。
这项研究表明,在岩心栓缺失的情况下,NMR测井和MDT 数据及电阻率测井相结合,提供了一种确定复杂岩性润湿性特性的方法。
引言润湿性表征了岩石被某一种流体相覆盖的相对偏向性。
显然,润湿性受岩石矿物和孔隙空间流体存在状态影响。
基于实测水压力的跨海桥梁围堰波浪力计算
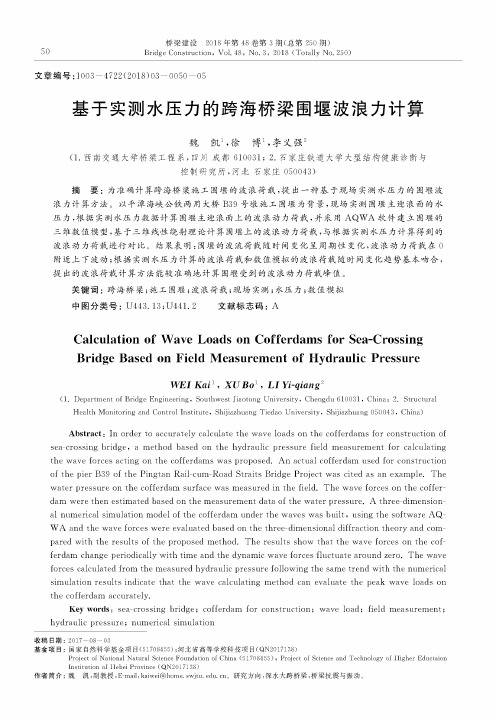
50桥梁建设2018年第48卷第3期(总第250期)Bridge Construction,Vol.48, No.3, 2018 (Totally No.250)文章编号:1003 —4722(2018)03 —0050 —05基于实测水压力的跨海桥梁围揠波浪力计算魏凯\徐博\李义强2(1.西南交通大学桥梁工程系,四川成都610031; 2.石家庄铁道大学大型结构健康诊断与控制研究所,河北石家庄050043)摘要:为准确计算跨海桥梁施工围堰的波浪荷载,提出一种基于现场实测水压力的围堰波 浪力计算方法。
以平潭海峡公铁两用大桥B3 9号墩施工围堰为背景,现场实测围堰主迎浪面的水压力,根据实测水压力数据计算围堰主迎浪面上的波浪动力荷载,并采用A Q W A软件建立围堰的三维数值模型,基于三维线性绕射理论计算围堰上的波浪动力荷载,与根据实测水压力计算得到的波浪动力荷载进行对比。
结果表明:围堰的波流荷载随时间变化呈周期性变化,波浪动力荷载在0附近上下波动;根据实测水压力计算的波浪荷载和数值模拟的波浪荷载随时间变化趋势基本吻合,提出的波浪荷载计算方法能较准确地计算围堰受到的波浪动力荷载峰值。
关键词:跨海桥梁;施工围堰;皮浪荷载;见场实测;水压力;数值模拟中图分类号:U443. 13;U441.2 文献标志码:ACalculation of Wave Loads on Cofferdams for Sea-CrossingBridge Based on Field Measurement of Hydraulic PressureWEI Kai1, XU Bo1, LI Yi-qiang2(1. Department of Bridge Engineering, Southwest Jiaotong University, Chengdu 610031, China;2. StructuralHealth Monitoring and Control Institute, Shijiazhuang Tiedao University, Shijiazhuang 050043, China)Abstract:In order t o accurately calculate the wave loads on the cofferdams for construction of sea-crossing bridge,a method based on the hydraulic pressure iield measurement for calculatingthe wave forces acting on the cofferdams was proposed.An actual cofferdam used for constructionof the pier B39 of the Pingtan Rai--cum-Road Straits Bridge Project was cited as an example.Thewater pressure on the cofferdam surface was measured in the tield.The wave forces on the cofferdam were then estimated based on the measurement data of the water pressure.A three-dimensional numerical simulation model of the cofferdam under the waves was built,using the software AQ-WA and the wave forces were evaluated based on the three-dimensional diffraction theory and compared with the r esults of the proposed method.The results show that the wave forces on the cofferdam change periodically with time and the dynamic wave forces fluctuate around forces calculated from the measured hydraulic pressure following the same trend with the numericalsimulation results indicate thatthe wave calculating method canevaluate the peak wave loads onthe cofferdam accurately.Key words:sea-crossing bridge;cofferdam for construction;wave load;tield measurement;hydraulic pressure;numerical simulation收稿日期:2017 — 08 — 03基金项目:国家自然科学基金项目(1708455);河北省髙等学校科技项目(QN2017138)Project of National Natural Science Foundation of China (51708455) ;Project of Science and Technology of Higher EductaionInstitution o flle b e i Province (QN2017138)作者筒介:魏凯,副教授,E-mail:kaiwei@home. sw 。
海洋环境中流体动力学的数值模拟方法
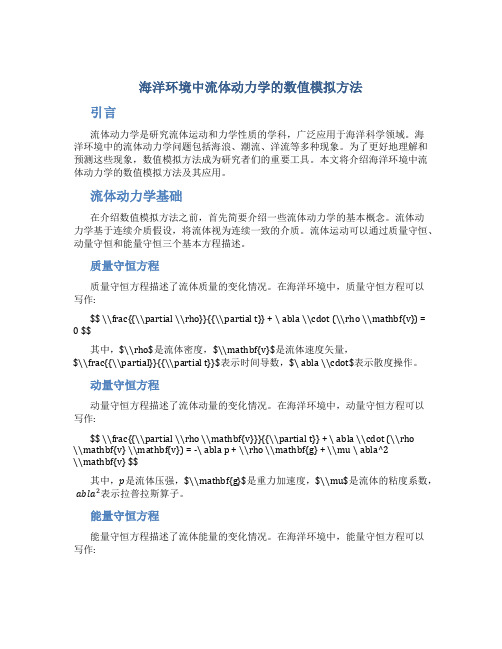
海洋环境中流体动力学的数值模拟方法引言流体动力学是研究流体运动和力学性质的学科,广泛应用于海洋科学领域。
海洋环境中的流体动力学问题包括海浪、潮流、洋流等多种现象。
为了更好地理解和预测这些现象,数值模拟方法成为研究者们的重要工具。
本文将介绍海洋环境中流体动力学的数值模拟方法及其应用。
流体动力学基础在介绍数值模拟方法之前,首先简要介绍一些流体动力学的基本概念。
流体动力学基于连续介质假设,将流体视为连续一致的介质。
流体运动可以通过质量守恒、动量守恒和能量守恒三个基本方程描述。
质量守恒方程质量守恒方程描述了流体质量的变化情况。
在海洋环境中,质量守恒方程可以写作:$$ \\frac{{\\partial \\rho}}{{\\partial t}} + \ abla \\cdot (\\rho \\mathbf{v}) = 0 $$其中,$\\rho$是流体密度,$\\mathbf{v}$是流体速度矢量,$\\frac{{\\partial}}{{\\partial t}}$表示时间导数,$\ abla \\cdot$表示散度操作。
动量守恒方程动量守恒方程描述了流体动量的变化情况。
在海洋环境中,动量守恒方程可以写作:$$ \\frac{{\\partial \\rho \\mathbf{v}}}{{\\partial t}} + \ abla \\cdot (\\rho\\mathbf{v} \\mathbf{v}) = -\ abla p + \\rho \\mathbf{g} + \\mu \ abla^2\\mathbf{v} $$其中,p是流体压强,$\\mathbf{g}$是重力加速度,$\\mu$是流体的粘度系数,abla2表示拉普拉斯算子。
能量守恒方程能量守恒方程描述了流体能量的变化情况。
在海洋环境中,能量守恒方程可以写作:$$ \\frac{{\\partial (\\rho E)}}{{\\partial t}} + \ abla \\cdot (\\rho \\mathbf{v} E) = -\ abla \\cdot (\\mathbf{v} p) + \ abla \\cdot (\\mu \ abla \\mathbf{v}) + \ abla \\cdot (\\mathbf{q} - \\mathbf{v} \\cdot \\mathbf{q}) $$其中,$\\rho E$是单位体积的总能量,$\\mathbf{q}$是热通量矢量。
稠油热化学驱渗流数学模型及数值模拟研究
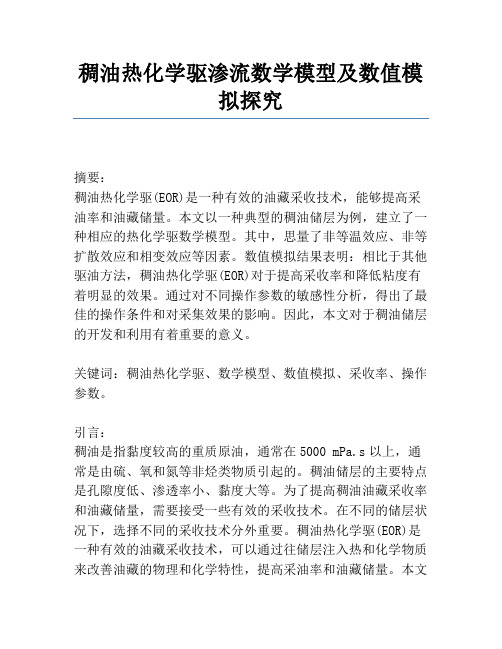
稠油热化学驱渗流数学模型及数值模拟探究摘要:稠油热化学驱(EOR)是一种有效的油藏采收技术,能够提高采油率和油藏储量。
本文以一种典型的稠油储层为例,建立了一种相应的热化学驱数学模型。
其中,思量了非等温效应、非等扩散效应和相变效应等因素。
数值模拟结果表明:相比于其他驱油方法,稠油热化学驱(EOR)对于提高采收率和降低粘度有着明显的效果。
通过对不同操作参数的敏感性分析,得出了最佳的操作条件和对采集效果的影响。
因此,本文对于稠油储层的开发和利用有着重要的意义。
关键词:稠油热化学驱、数学模型、数值模拟、采收率、操作参数。
引言:稠油是指黏度较高的重质原油,通常在5000 mPa.s以上,通常是由硫、氧和氮等非烃类物质引起的。
稠油储层的主要特点是孔隙度低、渗透率小、黏度大等。
为了提高稠油油藏采收率和油藏储量,需要接受一些有效的采收技术。
在不同的储层状况下,选择不同的采收技术分外重要。
稠油热化学驱(EOR)是一种有效的油藏采收技术,可以通过往储层注入热和化学物质来改善油藏的物理和化学特性,提高采油率和油藏储量。
本文的目标是建立一种数学模型来描述稠油热化学驱(EOR)过程,并进行数值模拟。
同时,本文通过对不同操作参数的敏感性分析,得出最佳的操作条件和对采集效果的影响。
模型:稠油热化学驱(EOR)是一个复杂的物理、化学和流淌过程,涉及到多个因素。
因此,建立一个综合思量了多种因素的数学模型分外重要。
在本文中,我们思量以下因素:1.热效应热效应是稠油热化学驱(EOR)的基本机理之一。
在注入高温液体后,油藏的温度会提高。
然后,由于油的黏度随温度提高而降低,油的流淌性得到提高,从而提高了采收率。
因此,我们思量不等温条件下的质量守恒方程和能量守恒方程来描述稠油油藏的流淌和热传递过程。
$$\begin{aligned} \frac{\partial \rho}{\partialt}+\nabla \cdot(\rho \mathbf{u}) &=0 (1) \\ \rho_{f} C_{p f} \frac{\partial T}{\partial t} &=\nabla\cdot(\lambda \nabla T)+H_{r e s}+Q-\rho C_{p f} u_{i} \frac{\partial T}{\partial x_{i}} (2) \\ \rho C_{pf}u&=-k \nabla p+\rho g+\mu \nabla^{2} u (3)\end{aligned}$$其中,(1)式为质量守恒方程,$\rho$为密度,$\mathbf{u}$为速度。
PO6016《湍流两相流动的模化与数值仿真》 课程教学大纲

2、掌握两相流的相似理论及模化方法,具备对两相流工程实际问题进行模 化设计与相似分析的能力。(A5.1、A5.4、B2、B4.1、B4.2)
的应用”和“基于数值仿真的热力新产品开发”,并开展课程陈述与讨论。通过面 向解决实际工程问题的课程实践,能够开拓学生的思路,教会他们运用理论知识 和科学的研究方法解决实际的科学技术问题,进行严格的科研训练和具备良好的 科研素质。
专题讲座 本课程将设置三次专业讲座,并通过工程案例分析具体讲解湍流两相流动的 模化方法和数值仿真技术,包括两相流模化方法、两相流数值仿真技术及其在工 程设计与产品开发中的应用。 四、考核与评估 课程得分比例如下:
1
课堂出席
10%
2
个人作业
15%
3 大作业(专题研究)
60%
4
课程陈述与讨论
15%
课堂出席 学生课堂出席成绩根据学生在课堂上的表现确定,包括出席、讨论、课堂练 习和表现等。评价课堂出席情况的标准包括参加者是否很好地倾听课程、能否积 极地参与课堂讨论、参加者的表达是否简洁和明确、能否有见地的分析案例和提 供清楚的论证。 个人作业 本课程在重点章节布置 4-6 次课后作业,主要是巩固已学基本概念和基本理 论,并运用基本知识解决关键问题,也推荐学生阅读经典的科技文献和综述。根 据作业完成情况和正确性评定成绩。 大作业(专题研究报告) 大作业(专题研究报告)是针对能源动力两相流工程实际问题开展专题研究,
出版商:
科学出版社
出版年:
1994
参考书目: Clement Kleinstreuer. Two-Phase Flow: Theory and Application, Taylor & Francis Group, New York, London, 2003 ISBN: 1-59169-000-5
铸铝ZL101与树脂砂型之间等效换热系数的数值模拟

铸铝Z L101 与树脂砂型之间等效换热系数的数值模拟薛祥, 周彼德, 蔺克亮(哈尔滨工业大学材料科学与工程学院, 黑龙江哈尔滨150001)摘要: 采用Z L01 铝合金作为成型材料,用呋喃树脂砂作为造型材料.采用24 通道的数据采集系统采集了铸件凝固与冷却过程中铸型、铸件内和铸件表面温度与时间的变化规律.从而为等效换热系数的反问题数值计算提供了第一手的数据.对两平板铸件进行了换热反问题的计算,其结果得出了两板件上下表面的等效换热系数K E 与时间的变化关系.可以看出等效换热系数在凝固刚开始时达到一个峰值,而后逐渐减小,并且其大小与铸件的横截面积有关,这些为今后数值模拟中确定等效热交换系数K E 提供了科学的计算数据.关键词: 换热系数; 传热反问题; 非线性估计法文章编号: 100520299 (2001) 022*******中图分类号: TG248 文献标识码:AN umerical simu lation of equivalent heat transfer coeff i cient bet w een ca s t al u minum all oy Z L101 and f uran resin sand mol dXUE Xiang , ZH OU Bi2de , L I N K e2liang( S chool of Materials S cience and Eng ineering , Harbin Institu te of Technolog y , Harb in 150001 , C hina)Abstract : In this study the aluminum all oy Z L101 was used as the casting m aterial and the furan resin sand was used as the m old m aterial . During the casting process , a 24 - channel com puter data acquisiti on system was used t o collect the tem perature values of the m olds , the castings and the surfaces of castings at di fferent tim es , which give the first - hand data for the inverse calculati on. Based on the software system , the tem perature values of tw o thick plane castings during solidi ficati on and cooling process were calculated and the values of equivalent heat transfer co2 efficient K E on the upper and l ower surfaces of the castings under di fferent tem peratures have also been com puted through sim ulati on. The diagram gives out the relati onship between K E and T . At the beginning of solidificati on , the K E reaches a peak value and then gradually decreases. The peak value is related to the cross secti on area of the castings. These results are helpful to understand the equivalent heat transfer coefficient during casting process and can play the role of a base for simulati on process.K ey w or d s : equivalent heat transfer coefficient ; inverse heat transfer problem ; num erical simulati on ; nonlinear es2 tim ati on technique在应用计算机模拟金属凝固热传输的研究中,铸件/ 铸型(冷铁) 界面换热条件的处理极大地影响了整个模拟结果,为此人们对铸件/ 铸型( 冷铁) 界面进行了研究,并提出了一些计算界面换热系数的方法 1 ~3 . 铸件/ 铸型界面上的传热是一个非常复杂的过程,在许多的情况下,界面换热往往是整个系统传热的关键. 大多数合金凝固时在铸件和铸型之间要形成气隙,因此引起界面热阻的变化,成为影响铸件凝固过程的重要因素1 . 尽管已有许多的学者在这方面作了研究,但至今仍没有一种令人满意的方法来确定界面热交换系数( K E) 值的大小4 . 可以看出对铸件进行凝固过程数值模拟时必须解决铸件与铸型之间的界面热交换问题. 但影响界面热交换的因素很多,例如铸件材质、几何形状、尺寸大小、凝固初始条件等. 界收稿日期: 2000 - 12 - 19 .作者简介: 薛祥(1961 - ) ,男,副教授,博士.第 2 期薛 祥 ,等 :铸铝 Z L101 与树脂砂型之间等效换热系数的数值模拟·207 ·1 .2 数据采集系统采用的温度测试装臵为 24 通道数据采集系 统 ,它可进行最多 24 点的连续采集 ,最近两点采 集时间间隔为 0 . 04 s.1 . 3 热电偶的排布考虑到整个铸件和铸型是一个很大的平面 , 在平面中心附近选了两个比较靠近的两点 ,这两 点代表其平面内的各点温度 . 为了能够在铸件及 铸型中方便的安装热电偶及准确的测量出热电偶 热点 的 位 臵 , 本 实 验 采 用 事 先 制 作 的 75 mm ×75 mm ×100 mm 砂块 ,把热电偶放在一个砂块中 ,水平与垂直方向共放臵 4 个热电偶 ,其摆放方式 如图 2 所示. 在随后的造型中把砂块先与模具贴 实后造型 ,就可以把砂快和砂型连为一体. 热电偶 直径为 0 . 2 mm ,为了增大敏感系数 ,铸型中热电 偶应尽可能的靠近铸件表面 . 将热电偶植入离铸 件表面几毫米的地方 ,只有利用上述方法才有效 . 在安装砂块的表 面 形 状 必 须 同 铸 件 的 轮 廓 相 符 合 . 本实验所测铸件是一个简单的平板状 ,所以它 的表面只需平面即可 . 砂块与铸型的材料相同 . 水 平热电偶在制造砂块的过程中放入离表面适当深 度的位臵 ,其竖直的两个热电偶在树脂砂没有变 硬之前先行插入.1 . 4 铸件表面温度测量为了能够精确的测量出平板表面的温度 ,首 先采用一个简单 的 双 点 热 电 偶 并 把 它 装 在 砂 块 中 . 从图 2 的侧视图 1 中看到 :一个热电偶是测量 铸件内部的温度的 ,而另一个热电偶是头部断开 的 (双点热电偶) ,是为了测量液面处的温度值. 其 原理可以用“中间金属定律”来解释7 ,8 ,11 . 本文把两个热电偶线 插 入 到 铸 型 型 腔 中 来 实 现 一 个 双 点热电偶 ,其尺寸如图2所示 . 当砂块装入铸面换热系数是一个综合反映这些因素随凝固过程 变化的参数 . 所以换热系数是一个十分难于把握的参数 ,但是它对铸件质量的影响十分巨大 ,因此 迫切需要解决此问题 .本文将通过实验测试的温度数据与传热的数 值模拟结合起来进行反问题的数值计算 ,从而建 立起各种条件下的换热系数与时间的关系曲线 , 该曲线能清晰的反映出不同条件 、不同时间的换 热系数 ( K E ) 的计算值 5 ~9证 .,并将此值经过实验验1 实验及数值计算方法1. 1 实验铸件铸造工艺本文所计算的铸件是平板铝合金铸件 ,合金 牌号为 Z L101 . 其尺寸分别为 200 mm ×200 mm ×25 mm 和 200 mm ×200 mm ×6 . 5 mm. 造型材料为 自硬呋喃树脂砂 . 原砂为 70/ 120 # 的 水 洗 硅 砂 , 呋喃树脂 ,粘接剂的加入量为原砂的 1 % ( 质量分 数) ,铸型系统如图 1 所示.图 1 铸型系统剖面图Fig. 1 S ection of pouring system图 2 电偶位臵尺寸图Fig. 2 Diag ram of location and d imension of therm ocou ples therm ocou ples中后 ,将这两个热电偶线伸入型腔中 . 熔化金属浇 注到型腔中时电流接通 ,所测得的温度是表面的 平均温度 ,其温度是第一个接触点的温度 ,伸入到平板中的电偶线对温度没有影响 . 1. 5 计算等效换热系数的数学模型K E 是一个时间函数. 它可以通过铸件凝固过·208 ·材 料 科 学 与 工 艺 第 9 卷程中实 测 铸 件 和 铸 型 内 部 温 度 变 化 的 一 系 列 数 值 ,用非线性估计法和数值法求解得到 . 非线性估 计法的 关 键 是 如 何 使 下 式 表 示 的 函 数 取 最 小 的 值 .根 据 前 面 的 数 值 模 型 及 其 一 系 列 的 约 束 条 件 ,本文应用 VC + + 可视化编程语言和面向对象 的编程技术对 C AS M - 3D 系统进行了补增 ,采用 非线性估计法为 C AS M - 3D 系统添加了等效换热 系数计算的功能. 尤其在界面处理方面做了大量 的工作 ,能够模拟出换热系数与时间的关系曲线 , 同时对 C AS M - 3D 系统在软件的可用性 、集成性 、 界面友好等方面做了一定的工作 . 从而进一步完 善了 C AS M - 3D 系统的功能. 选择了三点的温度 与时间关系曲线表示如图 3 和图 4 .n nT mij ]2f ( K E ) =∑∑[ T ij ( K E ) - i = 1 j = 1式中 : T mij 为 i 点 j 时刻的实测温度 , ℃; T ij ( K E ) 为 i 点 j 时刻的计算温度. ℃T ij ( K E ) ≈ T ij ( K E 1) + T ′i ΔjK E 1 +1 5 T ij ( K E )T ′i j =5 E 13 模拟结果及分析计算过程是以实验数据为基础进行的. 其吻 通过用非线性估计法 ,求出等效换热系数在 随时间变化时 ,一时间段内变化值与前时间段的 比值 . 当 小 于 一 个 规 定 的 小 数 时 就 可 以 确 定合程度与所选的限定小数 ( E ) 有关. 计算数据与 实验数据其吻合程度越好 , 其计算出来的等效换 热系数就越能代表各种影响因素 . 所以在计算时尽可能取 E 为一个非常小的数 . 但是这是以牺牲 计算时间为代价的 , 不过这样可以使计算的精度 大大的提高 . 而本文所计算的只是一个等效换热 系数与时间的一个关系 , 精度只要满足工业生产 的精度就可以了. 所以本文所采用的 E 取 0. 005.平板件几何形状非常简单 ,但其凝固及冷却 过程中的界面处的等效换热系数变化十分复杂 . 本文研究平板铝合金件凝固及冷却过程中界面处 等效换热系数的变化 ,是具有一定代表性的 . 铸件 材质为 Z L101 . 铸型为树脂砂 (1 % (质量分数) 呋喃 树脂) ,其热物性参数为模拟前确定. 剖分后网格 单元总数为 281 250 个 ,25 mm 厚板铸件单元 2 250 个 ,网格尺寸为 4 mm ×4 mm ×4 mm. 6 . 5 mm 厚板 铸件 单 元 数 为 750 , 网 格 尺 寸 为 4 mm ×4 mm ×2 mm ,铸型初始 温 度 为 18 ℃( 薄 板) 和 15 ℃( 厚 板) . 厚板件金属浇注温度为 690 ℃,薄板件的浇注温度为 716 ℃,铸型的初始温度都为 15 ℃,浇 口杯始终为充满状态 . 图 5 给出通过逆算法与非 线性估计法相结合而算出的两不同厚度的平板界面自等效换热系数与时间的关系 ,在其它的图中 给出放臵热电偶的点上的实测温度值与计算值的 比较 ,可以看出其吻合程度很好 ,精度也很高 .通过对计算模拟出来的等效换热系数与时间 的关系图分析可以得出金属液刚浇入型腔时 K E 迅速达到一个峰值 . 这是由于刚浇入时金属液与 铸 型表面具有良好固液接触所致 . 在图5 ( a ) 中换 热系数值 大 约 在 50 ~ 150 W/ m 2 ·℃的 范 围 之 内 . 在刚刚浇入后达到峰值 ,接下来后上下表面的换 热 系数产生一致的衰减 . 在图5 ( b ) 中换热系数值K El 4 ,5.nm∑∑[ T mij -T ij ( K E 1) ] T ′i ji = 1 j = 1ΔK E 1 =nm∑∑( T ′i j) 2i = 1 j = 1将上式用台劳级数展开 ,通过迭代过程使其 最小 . 迭代从估计一个 K E 1 开始. 对 I = 0 , 逐渐增 加迭代次数 , 直到Δ K E 1/ K E 1 < E ( E 为一个有限小的数) 便认为满足要求 , 可以转入下一个时间间隔 的 的 K E . 依次求出各时刻的 K E , 绘制出各种条件下 K E - t 的关系曲线 .2 实验结果的数据处理通过对数据进行分析可以得出 : 铸件的上下 表面的温度是一致的 . 在某些点处因为外界因素 的影响 ,可能造成一点差别. 但可以看出在铸型中 的热电偶所测出的数据有着很大的差别 . 在实验 准备时 ,热电偶埋在铸型中的深度是相同的. 但在 铸件冷却后 ,对铸型进行挖掘发现 ,埋在铸型中的 热电偶与铸件的距离发生了变化 . 其变化的具体 数据如表 1 所示 ,从中可以看出下铸型中的热电 偶位臵变化较大 ,而上铸型中的热电偶的位臵变 化较小.表 1 浇注前后铸型中热电偶位置变化T a b le1 Location chang es of thermocoup les after pouring25 mm 厚板 6. 5 mm 厚板型 体上型下型上型下型浇注前 浇注后5 57 85 77 95 57 95 67 7第 2 期薛 祥 ,等 :铸铝 Z L101 与树脂砂型之间等效换热系数的数值模拟·209 ·图 3 6. 5 mm 薄板铸件各点温度测量数值(a) 上表面 ( b ) 下表面(c) 铸件内 ( d ) 铸型中Measured resu lts of temperatures at d ifferen t points for the plane w ith 6. 5 mm thicknessFig. 3 图 4 25 mm 厚板件铸件温度测量数值(a) 上下表面 ( b ) 铸件内 (c) 铸型内Fig. 4 Measured resu lts of temperatures at d ifferen t p oints for theplane w ith 25 mm thickness为 50~300W/ m 2·℃,并且底部的换热系数值一直很高 ,这是由于下表面上的由于重力等原因使热 接触点 比 上 表 面 的 接 触 情 况 好 . 可 以 看 出 在 达 到 峰值后其值迅速下降 ,这是由于从固液接触转·210 ·材 料 科 学 与 工 艺 第 9 卷为固固接触和固液接触 . 从图 5 ( b ) 中还可以看出 上界面处的换热系数在 600 s 处有一个峰值 ,这表 明了平板铸件和铸型之间的热接触点的相对增加 了 . 上表面的热交换系数在达到一个峰值后马上 降到了一个比较平稳的值 ,这可能是由于处于上 表面与铸型间形成较明显的气隙所致 .分析图 5 可以明显的看出试样的截面尺寸及 位臵对换热系数有重要的影响 ,25 mm 厚的铸件 的下表面的换热系数近似是 6 . 5 mm 厚铸件的换 热系数的两倍 ,这一结果与国外的相关报导相同 .4 结 论(1) 采用逆算法与非线性估计法作为基础 .对界面处等效换热系数进行模拟计算. 得出界面 处等效换热系数与时间的关系图 ;(2) 分析了计算模拟结果所得出的曲线. 发 现界面换热系数与铸件的截面尺寸有着十分密切 的关系 ,随着界面尺寸的增加 ,其等效换热系数加 大 .参考文献 :1WOODBURY K A , CHEN Y , PARKER J K , et al . Mea 2 surement of heat 2trans fer coefficien ts b etw een Al castings and resin 2b on d ed M olds J . AFS Transactions , 1998 , (116) : 7052711. 程 军 , 柳百成 . 铸件 - 铸型界面热交换参数的通 用化处理 J .铸造 , 1991 , (2) : 22225.2 3 李德林 , 周尧和 . 铸件/ 冷铁界面换热研究 J . 属科学与工艺 , 1990 , (6) : 53257.金4 高尚书 . 计算机技术及其在铸造行业中的应用 J . 铸造 , 1995 , (6) : 31237.曾松岩 , 王深强 , 蒋祖令 , 等 . 铸件物性值的测定方 法及计算机辅助校正 J . 铸造 , 1989 , (5) : 16221.佟天夫 , 张大辉 . 熔模铸造金属与型壳界面作用的研究 J .铸造 , 1993 , (2) : 126.HO , K , PEH L KE R D. Transient m ethods for d etermina 2 tion of metal 2m old interfacial heat trans fer J . AFS Trans 2 actions , 1983 , (80) : 6892698.HO K , PEH L K E R D. Mechanisms of heat trans fer at a metal 2m old interface J . AFS Transactions , 1984 , (61) : 5872597.袁浩扬 , 闵光国 , 林汉同 , 等 . 凝固模拟中实用三维 几何造型方法的研究 J . 铸造 , 1994 , (2) : 8211. 第五十届国际铸造会议论文摘要 . 铸造 . 1984 : 45248.(责任编辑 张积滨)5 6 78 图 5 界面等效热交换系数与时间变化关系(a ) 6. 5 mm 厚板件( b ) 25 mm 厚板件9 Fig. 5Relationship b etw een equ ivalent heat trans fer coefficientand time10。
基于CFD方法对摆线推进器水动力性能的数值预报
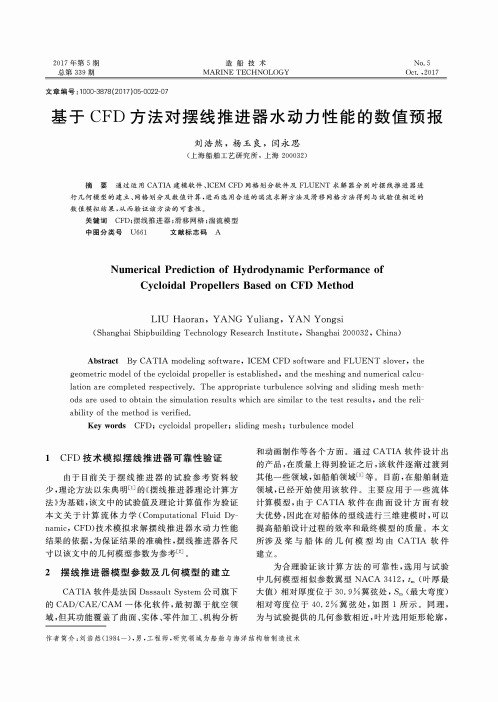
2017年第5期 总第339期造船技术MARINE TECHNOLOGYNo.5Oct.,2017文章编号:1〇〇〇-3878 (2017) 〇5-〇022-〇7基于C F D方法对摆线推进器水动力性能的数值预报刘浩然,杨玉良,闫永思(上海船舶工艺研究所,上海200032)摘要通过运用C A T IA建模软件、IC E M C FD网格划分软件及F L U E N T求解器分别对摆线推进器进行几何模型的建立、网格划分及数值计算,进而选用合适的湍流求解方法及滑移网格方法得到与试验值相近的数值模拟结果,从而验证该方法的可靠性。
关键词CFD;摆线推进器;滑移网格;湍流模型中图分类号U661 文献标志码 ANumerical Prediction of Hydrodynamic Performance ofCycloidal Propellers Based on CFD MethodLIU Haoran,YA N G Yuliang,Y A N Yongsi(Shanghai Shipbuilding Technology Research Institute,Shanghai 200032,China)Abstract By CATIA modeling software,ICEM CFD software and FLU EN T slover,the geometric model of the cycloidal propeller is established?and the meshing and numerical calculation are completed respectively.The appropriate turbulence solving and sliding mesh methods are used to obtain the simulation results which are similar to the test results,and the reliability of the method is verified.Key words CFD;cycloidal propeller;sliding mesh;turbulence model1 C F D技术模拟摆线推进器可靠性验证由于目前关于摆线推进器的试验参考资料较 少,理论方法以朱典明[1]的《摆线推进器理论计算方 法》为基础,该文中的试验值及理论计算值作为验证 本文关于计算流体力学(Computational Fluid Dynamic,CFD)技术模拟求解摆线推进器水动力性能 结果的依据,为保证结果的准确性,摆线推进器各尺 寸以该文中的几何模型参数为参考[2]。
太阳能溶液集热_再生器数值模拟分析_魏昊然
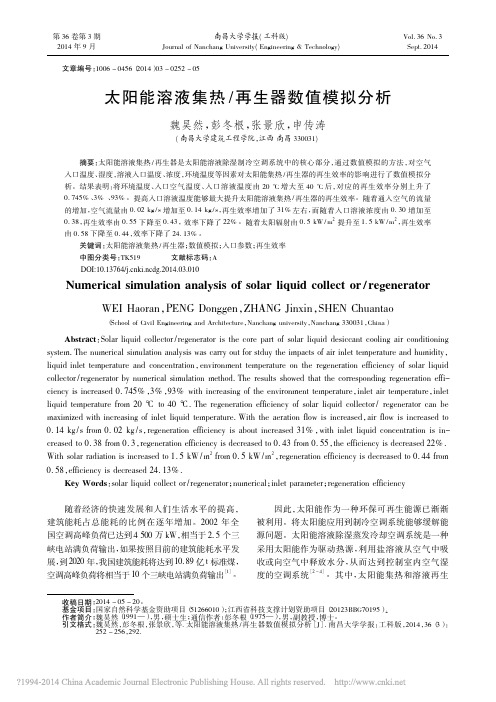
收稿日期:2014-05-20。
基金项目:国家自然科学基金资助项目(51266010);江西省科技支撑计划资助项目(20123BBG70195)。
作者简介:魏昊然(1991—),男,硕士生;通信作者:彭冬根(1975—),男,副教授,博士。
引文格式:魏昊然,彭冬根,张景欣,等.太阳能溶液集热/再生器数值模拟分析[J ].南昌大学学报:工科版,2014,36(3):252-256,292.文章编号:1006-0456(2014)03-0252-05太阳能溶液集热/再生器数值模拟分析魏昊然,彭冬根,张景欣,申传涛(南昌大学建筑工程学院,江西南昌330031)摘要:太阳能溶液集热/再生器是太阳能溶液除湿制冷空调系统中的核心部分,通过数值模拟的方法,对空气入口温度、湿度,溶液入口温度、浓度,环境温度等因素对太阳能集热/再生器的再生效率的影响进行了数值模拟分析。
结果表明:将环境温度、入口空气温度、入口溶液温度由20ħ增大至40ħ后,对应的再生效率分别上升了0.745%、3%、93%。
提高入口溶液温度能够最大提升太阳能溶液集热/再生器的再生效率。
随着通入空气的流量的增加,空气流量由0.02kg /s 增加至0.14kg /s ,再生效率增加了31%左右,而随着入口溶液浓度由0.30增加至0.38,再生效率由0.55下降至0.43,效率下降了22%。
随着太阳辐射由0.5kW /m 2提升至1.5kW /m 2,再生效率由0.58下降至0.44,效率下降了24.13%。
关键词:太阳能溶液集热/再生器;数值模拟;入口参数;再生效率中图分类号:TK519文献标志码:ANumerical simulation analysis of solar liquid collect or /regeneratorWEI Haoran ,PENG Donggen ,ZHANG Jinxin ,SHEN Chuantao(School of Civil Engineering and Architecture ,Nanchang university ,Nanchang 330031,China )Abstract :Solar liquid collector /regenerator is the core part of solar liquid desiccant cooling air conditioning system.The numerical simulation analysis was carry out for stduy the impacts of air inlet temperature and humidity ,liquid inlet temperature and concentration ,environment temperature on the regeneration efficiency of solar liquid collector /regenerator by numerical simulation method.The results showed that the corresponding regeneration effi-ciency is increased 0.745%,3%,93%with increasing of the environment temperature ,inlet air temperature ,inlet liquid temperature from 20ħto 40ħ.The regeneration efficiency of solar liquid collector /regenerator can be maximized with increasing of inlet liquid temperature.With the aeration flow is increased ,air flow is increased to 0.14kg /s from 0.02kg /s ,regeneration efficiency is about increased 31%,with inlet liquid concentration is in-creased to 0.38from 0.3,regeneration efficiency is decreased to 0.43from 0.55,the efficiency is decreased 22%.With solar radiation is increased to 1.5kW /m 2from 0.5kW /m 2,regeneration efficiency is decreased to 0.44from 0.58,efficiency is decreased 24.13%.Key Words :solar liquid collect or /regenerator ;numerical ;inlet parameter ;regeneration efficiency 随着经济的快速发展和人们生活水平的提高,建筑能耗占总能耗的比例在逐年增加。
水下爆炸冲击波数值仿真精度研究
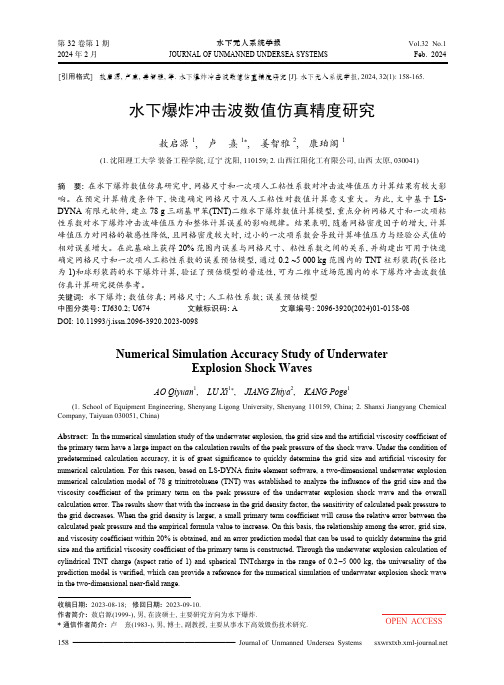
水下爆炸冲击波数值仿真精度研究敖启源 1, 卢 熹 1*, 姜智雅 2, 康珀阁 1(1. 沈阳理工大学 装备工程学院, 辽宁 沈阳, 110159; 2. 山西江阳化工有限公司, 山西 太原, 030041)摘 要: 在水下爆炸数值仿真研究中, 网格尺寸和一次项人工粘性系数对冲击波峰值压力计算结果有较大影响。
在预定计算精度条件下, 快速确定网格尺寸及人工粘性对数值计算意义重大。
为此, 文中基于LS-DYNA有限元软件, 建立78 g三硝基甲苯(TNT)二维水下爆炸数值计算模型, 重点分析网格尺寸和一次项粘性系数对水下爆炸冲击波峰值压力和整体计算误差的影响规律。
结果表明, 随着网格密度因子的增大, 计算峰值压力对网格的敏感性降低, 且网格密度较大时, 过小的一次项系数会导致计算峰值压力与经验公式值的相对误差增大。
在此基础上获得20%范围内误差与网格尺寸、粘性系数之间的关系, 并构建出可用于快速确定网格尺寸和一次项人工粘性系数的误差预估模型, 通过0.2 ~5 000 kg范围内的TNT柱形装药(长径比为1)和球形装药的水下爆炸计算, 验证了预估模型的普适性, 可为二维中近场范围内的水下爆炸冲击波数值仿真计算研究提供参考。
关键词: 水下爆炸; 数值仿真; 网格尺寸; 人工粘性系数; 误差预估模型中图分类号: TJ630.2; U674 文献标识码: A 文章编号: 2096-3920(2024)01-0158-08DOI: 10.11993/j.issn.2096-3920.2023-0098Numerical Simulation Accuracy Study of UnderwaterExplosion Shock WavesAO Qiyuan1, LU Xi1*, JIANG Zhiya2, KANG Poge1(1. School of Equipment Engineering, Shenyang Ligong University, Shenyang 110159, China; 2. Shanxi Jiangyang Chemical Company, Taiyuan 030051, China)Abstract: In the numerical simulation study of the underwater explosion, the grid size and the artificial viscosity coefficient of the primary term have a large impact on the calculation results of the peak pressure of the shock wave. Under the condition of predetermined calculation accuracy, it is of great significance to quickly determine the grid size and artificial viscosity for numerical calculation. For this reason, based on LS-DYNA finite element software, a two-dimensional underwater explosion numerical calculation model of 78 g trinitrotoluene (TNT) was established to analyze the influence of the grid size and the viscosity coefficient of the primary term on the peak pressure of the underwater explosion shock wave and the overall calculation error. The results show that with the increase in the grid density factor, the sensitivity of calculated peak pressure to the grid decreases. When the grid density is larger, a small primary term coefficient will cause the relative error between the calculated peak pressure and the empirical formula value to increase. On this basis, the relationship among the error, grid size, and viscosity coefficient within 20% is obtained, and an error prediction model that can be used to quickly determine the grid size and the artificial viscosity coefficient of the primary term is constructed. Through the underwater explosion calculation of cylindrical TNT charge (aspect ratio of 1) and spherical TNTcharge in the range of 0.2–5 000 kg, the universality of the prediction model is verified, which can provide a reference for the numerical simulation of underwater explosion shock wave in the two-dimensional near-field range.收稿日期: 2023-08-18; 修回日期: 2023-09-10.作者简介: 敖启源(1999-), 男, 在读硕士, 主要研究方向为水下爆炸.* 通信作者简介: 卢 熹(1983-), 男, 博士, 副教授, 主要从事水下高效毁伤技术研究.第 32 卷第 1 期水下无人系统学报Vol.32 N o.1 2024 年 2 月JOURNAL OF UNMANNED UNDERSEA SYSTEMS Feb. 2024[引用格式] 敖启源, 卢熹, 姜智雅, 等. 水下爆炸冲击波数值仿真精度研究[J]. 水下无人系统学报, 2024, 32(1): 158-165.Keywords: underwater explosion; numerical simulation; grid size; artificial viscosity coefficient; error prediction model0 引言水下武器作为舰船生命力的主要威胁之一, 其爆炸冲击波及气泡载荷会对舰船造成严重的局部和总体破坏[1]。
开设内槽翼型的水动力性能数值模拟

收稿日期:2019-09-30修回日期:2019-12-02网络首发时间:2020-7-299:41基金项目:国家自然科学基金资助项目(51679052);国家部委基金资助项目;国家重点实验室基金资助项目(6142223180210)作者简介:解学参,男,1982年生,博士,高级工程师。
研究方向:船舶总体研究与设计,船舶推进与节能技术。
E-mail :*******************常晟铭,男,1996年生,硕士生。
研究方向:船舶推进与节能技术。
E-mail :************************.cn 王超,男,1981年生,博士,副教授。
研究方向:船舶推进与节能技术。
E-mail :**********************.cn*通信作者:王超引言随着航运业的发展,对船舶的快速性以及操纵性能有了更高的要求。
水下翼型结构特点优越,利于船舶的减阻和节能,常用于舵和减摇鳍的设计之中。
舵作为船舶的操纵装置,其设计一方开设内槽翼型的水动力性能数值模拟解学参1,常晟铭2,王超*2,郭春雨21中国舰船研究设计中心,湖北武汉4300642哈尔滨工程大学船舶工程学院,黑龙江哈尔滨150001摘要:[目的]水下翼型具有减阻和节能的特点,因此,常应用于高性能船舶的舵和减摇鳍的设计之中。
从目前对翼型的水动力性能研究来看,对翼型进行一定程度的改进,如开设内槽、增设前缘结节等,均会使翼型的水动力性能得到显著提升。
为研究开设不同形式的内槽对翼型水动力性能的影响并探求相关作用机理,开展相关研究。
[方法]对NACA0012型号的翼型开设内槽,利用计算流体力学的方法对具有不同内槽形式的翼型进行水动力计算。
[结果]结果表明:将椭圆形式的内槽开设在翼型前缘对翼型水动力性能的提升最为显著,而为了保证内槽处水流的平稳流动,内槽应有一定的宽度。
[结论]研究成果能够对船用舵的改进起到一定的参考作用。
关键词:水翼;内槽;水动力性能;数值模拟中图分类号:U664.3文献标志码:ADOI :10.19693/j.issn.1673-3185.01789Numerical simulation of hydrodynamic performance of hydrofoil with inner grooveXIE Xuesen 1,CHANG Shengmin 2,WANG Chao *2,GUO Chunyu 21China Ship Development and Design Center ,Wuhan 430064,China 2College of Shipbuilding Engineering ,Harbin Engineering University ,Harbin 150001,China Abstract :[Objectives ]Underwater hydrofoils have the characteristics of drag reduction and energy conservation.They are often used in the design of rudder and fin stabilizers for high-performance ships.From current research on the hydrodynamic performance of hydrofoils ,such improvements as opening the inner groove and adding a leading edge nodule can significantly improve their hydrodynamic performance.[Methods ]In this paper ,an NACA0012hydrofoil with internal slots is improved ,the influence ofdifferent internal slots on the hydrodynamic performance of the hydrofoil is studied by means of computational fluid mechanics ,and the relevant action mechanism is explored.[Results ]Finally ,it is concluded that the hydrodynamic performance of the hydrofoil can be improved most significantly by placing the elliptical inner groove at the leading edge of the hydrofoil ,and the inner groove should have a certain width to ensure a smooth flow.[Conclusions ]The results of this study can play a certain role in the improvement of ship rudders.Key words :hydrofoil ;inner groove ;hydrodynamic performance ;numerical simulation引用格式:解学参,常晟铭,王超,等.开设内槽翼型的水动力性能数值模拟[J ].中国舰船研究,2020,15(4):127-134.XIE X S ,CHANG S M ,WANG C ,et al.Numerical simulation of hydrodynamic performance of hydrofoil with inner groove [J ].Chinese Journal of Ship Research ,2020,15(4):127-134.扫码阅读全文中国舰船研究第15卷面要考虑船舶的快速性而减小其阻力,另一方面也要考虑船舶的回转性,即能够在其有效舵角处提供较大的升力。
自流式空气除尘系统管道中过饱和度分布特征

第52卷第12期2021年12月中南大学学报(自然科学版)Journal of Central South University (Science and Technology)V ol.52No.12Dec.2021自流式空气除尘系统管道中过饱和度分布特征代安稳,张军,尹杰,叶晓辉(东南大学能源与环境学院,江苏南京,210096)摘要:为了探究自流式空气除尘系统的过饱和度,为系统优化提供依据,首先,简化自流式空气除尘系统,构建物理和数学模型;其次,使用Fluent 用户自定义标量(UDS)对水汽相变传热传质模型进行计算,研究自然对流情况下管道直径、进口空气温度、壁面热水温度、进口空气湿度及热水加入位置等因素对管道中过饱和度分布的影响;最后,使用响应面分析法进行三因素三水平单响应试验设计,确定管道直径、热水温度及热水加入位置对过饱和度的影响程度。
研究结果表明:随着管道直径增加,平均过饱和度呈现出先升高后降低的趋势;平均过饱和度随着空气与热水温差增大而增大;进口空气相对湿度对过饱和度的影响较小;相较于热水的全覆盖,热水加入位置靠近管道出口更有利于提高管道的过饱和度;热水温度对过饱和度影响要远大于管道直径与热水加入位置的影响,管道直径与热水加入位置对过饱和度影响相近。
在优化系统工况参数时,可着重考虑热水温度的影响,而将管道直径及热水加入位置作为次要因素考虑。
关键词:PM 2.5;过饱和度;水汽相变;Fluent 中图分类号:TK16文献标志码:A开放科学(资源服务)标识码(OSID )文章编号:1672-7207(2021)12-4252-10Distribution characteristics of supersaturation in pipeline ofgravity air dust removal systemDAI Anwen,ZHANG Jun,YIN Jie,YE Xiaohui(School of Energy and Environment,Southeast University,Nanjing 210096,China)Abstract:The supersaturation level of gravity air dust removal system was explored to provide a basis for system optimization.Firstly,the gravity air dust removal system was simplified and physical and mathematical models were constructed.Secondly,Fluent user-defined scalar(UDS)was used to analyze water vapor phase.The variable heat and mass transfer models were calculated.The influence of factors such as pipe diameter,inlet air temperature,wall hot water temperature,inlet air humidity and hot water adding position on the distribution of supersaturation in the pipe during natural convection was studied.Finally,a three-factor three-level single-response test design was carried out using response surface analysis to determine the degree of influence of pipe收稿日期:2021−03−10;修回日期:2021−05−18基金项目(Foundation item):国家自然科学基金资助项目(51876038)(Project(51876038)supported by the National Natural ScienceFoundation of China)通信作者:张军,男,博士,教授,从事燃煤污染控制研究;E-mail :****************.cnDOI:10.11817/j.issn.1672-7207.2021.12.006引用格式:代安稳,张军,尹杰,等.自流式空气除尘系统管道中过饱和度分布特征[J].中南大学学报(自然科学版),2021,52(12):4252−4261.Citation:DAI Anwen,ZHANG Jun,YIN Jie,et al.Distribution characteristics of supersaturation in pipeline of gravity air dust removal system[J].Journal of Central South University(Science and Technology),2021,52(12):4252−4261.第12期代安稳,等:自流式空气除尘系统管道中过饱和度分布特征diameter,hot water temperature and hot water addition position on supersaturation.The results show that as the pipe diameter increases,the average supersaturation increases firstly and then decreases.The average supersaturation increases with the increase of the temperature difference between air and hot water.The relative humidity of the inlet air has little effect on the degree of pared with the full coverage of hot water,it is more beneficial to increase the supersaturation level of the pipeline when the hot water is added to the location close to the outlet of the pipeline.The hot water temperature has a much greater impact on the supersaturation than the diameter of the pipeline and the location of hot water.The diameter and the position where the hot water is added have the same effect on the supersaturation.When optimizing the system working condition parameters,the influence of hot water temperature can be emphatically considered and the pipe diameter and hot water adding position can be considered as secondary factors.Key words:PM2.5;supersaturation;vapor phase transition;FluentPM2.5是雾霾的重要成分,控制PM2.5仍是环境领域研究的热点[1−3]。
水平管流中水合物颗粒运移和沉积数

价值工程0引言水合物流动保障问题已成为制约深水油气开发的关键问题之一[1]。
深海浅部地层具有低温高压环境,容易在生产管柱中生成水合物,沉积并附着在管壁上,导致井筒出现水合物堵塞风险。
研究水合物颗粒的运移和沉积规律对探究水合物堵塞的机理具有重要意义。
Lingelem 等人(1994)提出了天然气主导体系中水合物生成沉积的概念机理,在初始结晶阶段,水分子和气体分子分散在冷管管壁,形成亚临界核,进而生长为晶体;随着水分子与气体分子不断扩散,晶体持续生长,最终形成水合物堵塞[2]。
Turner 等人(2005)针对气液共存系统建立了水合物动力学模型:CSMHyK 模型,将过冷度和气液接触面积考虑在内[3]。
Jassim 等人(2010)使用CFD 技术模拟了单水合物颗粒沉积的实验,并提出了一种计算气主导系统中单水合物颗粒沉降的理论模型[4]。
Di Lorenzo 等人首次对管道内环形雾流中的水合物形成和输送进行了室内实验研究,并获得了大量实验数据,如水合物形成速率、管壁上的水合物层生长速率等[5]。
Wang 等人讨论了环雾流中的水合物沉积特征,并初步发现,气芯中液滴产生的水合物颗粒和管壁液膜处产生的水合物颗粒均会沉积并粘附在管壁上[6]。
目前少有研究关注水合物颗粒在气相中在管壁附近的运动状态。
本文利用优化的DPM 模型模拟了水合物颗粒在气芯中的运移和沉积特征,得到了水合物颗粒的运移和沉降特征,为准确预测水合物沉积堵塞提供了理论依据。
1求解模型气井井筒以环雾流为主,气相连续分布,液相以管壁液膜与气核液滴形式存在,两种形式均为水合物生成创造了条件,如图1所示。
研究该体系水合物沉积机理,必须综合考虑液膜与气核液滴中颗粒群的沉积。
液膜中由于自由水存在,颗粒与壁面间的粘附力很大,水合物将直接沉积于壁面,前人对此研究做的工作较充分,本文主要针对气核液滴中的水合物颗粒沉积机理展开研究。
本文采用的数值模型主要是离散相模型(DPM ),颗粒力平衡方程的形式由公式(1)表示,该式右边第一项为颗粒单位质量力,第二项是重力项,第三项是粒子其他力[7],水合物颗粒受力示意图见图2。
《基于拉瓦尔效应的气水两相喷雾数值模拟研究》范文
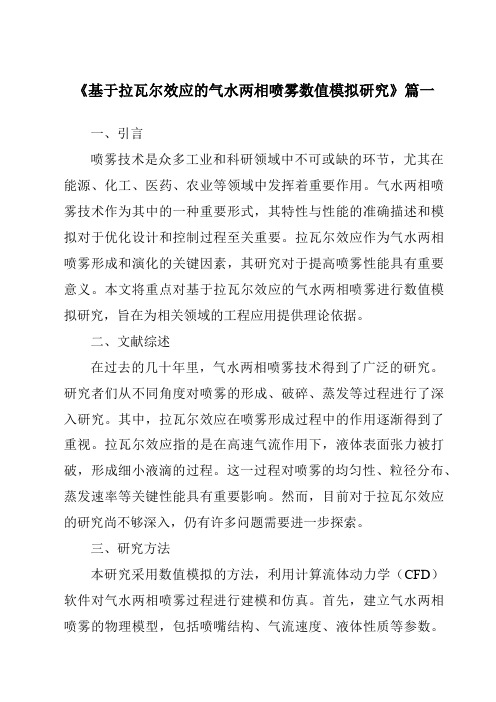
《基于拉瓦尔效应的气水两相喷雾数值模拟研究》篇一一、引言喷雾技术是众多工业和科研领域中不可或缺的环节,尤其在能源、化工、医药、农业等领域中发挥着重要作用。
气水两相喷雾技术作为其中的一种重要形式,其特性与性能的准确描述和模拟对于优化设计和控制过程至关重要。
拉瓦尔效应作为气水两相喷雾形成和演化的关键因素,其研究对于提高喷雾性能具有重要意义。
本文将重点对基于拉瓦尔效应的气水两相喷雾进行数值模拟研究,旨在为相关领域的工程应用提供理论依据。
二、文献综述在过去的几十年里,气水两相喷雾技术得到了广泛的研究。
研究者们从不同角度对喷雾的形成、破碎、蒸发等过程进行了深入研究。
其中,拉瓦尔效应在喷雾形成过程中的作用逐渐得到了重视。
拉瓦尔效应指的是在高速气流作用下,液体表面张力被打破,形成细小液滴的过程。
这一过程对喷雾的均匀性、粒径分布、蒸发速率等关键性能具有重要影响。
然而,目前对于拉瓦尔效应的研究尚不够深入,仍有许多问题需要进一步探索。
三、研究方法本研究采用数值模拟的方法,利用计算流体动力学(CFD)软件对气水两相喷雾过程进行建模和仿真。
首先,建立气水两相喷雾的物理模型,包括喷嘴结构、气流速度、液体性质等参数。
其次,运用拉瓦尔效应理论,分析喷雾形成过程中的流体动力学特性。
最后,通过CFD软件对模型进行求解,得到喷雾的粒径分布、速度场、温度场等关键参数。
四、结果与讨论1. 喷雾形态与粒径分布模拟结果显示,在拉瓦尔效应的作用下,气水两相喷雾形成了均匀、细小的液滴。
随着气流速度的增加,液滴的粒径逐渐减小,喷雾的均匀性得到提高。
这表明拉瓦尔效应有助于改善喷雾的质量。
2. 速度场与温度场在拉瓦尔效应的影响下,喷雾的速度场和温度场呈现出明显的变化。
速度场显示,喷雾核心区域的流速较高,随着距离的增加,流速逐渐降低。
温度场则表明,在喷雾过程中,液滴的蒸发导致温度逐渐升高。
这些结果为进一步优化喷雾过程提供了重要依据。
3. 影响因素分析本研究还探讨了不同因素对气水两相喷雾的影响。
《2024年基于拉瓦尔效应的气水两相喷雾数值模拟研究》范文

《基于拉瓦尔效应的气水两相喷雾数值模拟研究》篇一一、引言随着现代工业和能源技术的不断发展,气水两相喷雾技术已成为众多领域中不可或缺的关键技术之一。
拉瓦尔效应作为气水两相喷雾技术的重要理论基础,其研究对于提高喷雾效率、优化能源利用等方面具有重要意义。
本文旨在通过数值模拟方法,对基于拉瓦尔效应的气水两相喷雾进行深入研究,以期为相关领域的技术研发和应用提供理论支持。
二、文献综述在过去的研究中,气水两相喷雾的数值模拟已经取得了丰硕的成果。
在探讨喷雾过程中,拉瓦尔效应扮演着至关重要的角色。
拉瓦尔效应即通过喷嘴将高压气体与液体混合并加速,从而在喷嘴出口形成超音速喷雾。
相关研究表明,合理的喷嘴设计和操作条件对于提高喷雾效率、优化能源利用具有重要意义。
然而,现有的研究仍存在一些不足。
例如,在气水两相喷雾的数值模拟中,对喷嘴内部流场、喷雾形态、雾化效果等方面的研究还不够深入。
此外,针对不同工况下的喷雾特性及影响因素的研究也相对较少。
因此,本文将基于拉瓦尔效应,对气水两相喷雾进行更深入的数值模拟研究。
三、研究内容1. 模型建立与假设本研究采用数值模拟方法,建立气水两相喷雾的物理模型。
假设喷嘴内部流场为稳态、一维流动,忽略喷嘴内部的热传导和热辐射效应。
同时,考虑到实际工况的复杂性,对喷雾过程进行合理的简化和假设,以便于数值模拟的实现。
2. 数值方法与软件本研究采用计算流体动力学(CFD)方法进行数值模拟。
选用合适的湍流模型、多相流模型以及喷雾模型等,以描述气水两相喷雾的流动特性和雾化效果。
利用专业的CFD软件进行建模、求解和后处理,以获得喷雾过程的详细信息。
3. 喷嘴设计与操作条件喷嘴的设计和操作条件对于气水两相喷雾的特性具有重要影响。
本研究将设计不同结构参数的喷嘴,如喷孔直径、喷孔数量、喷孔间距等,以探究其对喷雾效果的影响。
同时,还将研究操作条件(如气体压力、液体流量等)对喷雾特性的影响。
4. 模拟结果与分析通过数值模拟,获得气水两相喷雾的流场分布、喷雾形态、雾化效果等详细信息。
水下爆炸柱形高压气泡膨胀过程的RGFM和高精度格式数值模拟

水下爆炸柱形高压气泡膨胀过程的RGFM和高精度格式数值模拟史汝超;张亚军;徐胜利【摘要】为了对柱形装药水下爆炸高压气泡膨胀过程进行三维数值模拟,用level set方法追踪气水界面,详细描述了精确对柱形气泡进行level set建模;对于流场,使用Euler方程描述,并用高精度格式(五阶WENO和四阶R-K法)离散空间项和时间项;对于level set方程,使用五阶HJ-WENO离散;用RGFM处理气水界面附近网格节点.给出了水下流场不同时刻的压力云图、柱形高压气泡的形状演变以及流场中几个指定点的压力峰值.通过三维建模和计算验证,用RGFM结合高精度格式可以很好地对柱形高压气泡膨胀问题进行三维数值模拟,同时也可以较精确地追踪高密度比、高压力比的三维气水界面.计算结果表明,柱形高压气泡在膨胀过程中,形状逐渐向椭球形变化;位于固壁附近的柱形高压气泡受固壁反射波的影响,在固壁法线方向上的膨胀会受到抑制;双圆柱形高压气泡膨胀产生的冲击波,可以彼此抑制对方的膨胀.【期刊名称】《爆炸与冲击》【年(卷),期】2014(034)004【总页数】5页(P439-443)【关键词】爆炸力学;高压气泡膨胀;level set方法;水下爆炸;高精度格式;虚拟流体方法【作者】史汝超;张亚军;徐胜利【作者单位】中国科学技术大学近代力学系,安徽合肥230026;中国工程物理研究院流体物理研究所,四川绵阳621999;中国科学技术大学近代力学系,安徽合肥230026【正文语种】中文【中图分类】O382.1柱形高压气泡膨胀问题常见于柱形装药水下爆炸。
对此类问题进行数值模拟,精确处理和追踪高压力比、高密度比的气水界面一直是难点。
Rayleigh-Plesset运动方程[1]为众多气泡运动理论研究提供了参考;J.R.Blake等[2]和A.Pearson等[3]采用边界元方法研究了水下爆炸气泡演化过程;T.A.Vernon[4]和Z.Zong[5]采用拉普拉斯方程描述速度场,研究高压气泡运动。
HydroGeoSphere在锦屏水电站坝址区水流和溶质运移模拟中的应用

黄勇,等:HydroGeoSphere在锦屏水电站坝址区水电站坝址区水流和溶质运移模拟中的应用2431 引言裂隙岩体主要由岩体基质和裂隙网络组成,岩体基质以贮水为主,通常采用等效连续介质模型描述;裂隙网络以导水为主,常采用离散网络模型描述。
模型的求解主要采用有限体积法,如Therrien 和Sudicky[1],Graf和Therrien[2], Bodin等[3], Bodin等[4], Peratta等[5]和Ito等[6]分别采用有限体积法对连续-离散裂隙耦合模型进行了研究,刘玉玲[7]将基于流向量分裂技术的两步有限体积分量方向TVD格式应用于求解浅水方程和污染物输运方程,建立了二者耦合求解的高分辨率计算模型。
基于有限体积法,He Feng-yun[8]对非稳定粘性流体的方程进行了离散。
此外,McKennaa等[9]将两种不同的随机模型应用在Yucca Mountain地区的溶质运移模拟,获得了很好的结果。
何杨等(2007) [10]针对稀疏的三维主干岩体裂隙网络,以渗流区内的水量平衡原理为依据建立基本方程,推导三维岩体裂隙网络非稳定渗流的基本方程,并利用Monte-Carlo模拟了岩体中的裂隙网路。
Zhang Qian-fei(2008)[11]利用欧拉-拉格朗日方法对裂隙岩体中的水流和对流-弥散过程进行了研究,Li Min(2007)使用积分变换法获得了承压含水层中地下水流运移规律的解析解[12]。
裂隙岩体中渗透参数和溶质迁移参数主要采用示踪试验获得(Weatherill和Salve[13,14])基于HydroGeoSphere软件包,本文采用等效连续介质模型和耦合模型来模拟裂隙岩体中的水流和溶质运移规律,含水层参数的率定主要利用实测的地下水位和示踪试验的结果,并对两种模型的模拟结果进行了对比。
2 地质概况锦屏Ι级水电站位于四川省的木里和盐源县境内,距雅砻江的下游出口约358 km,研究区域介于雅砻江、锦屏断层、普斯罗沟和手爬沟之间(图1),雅砻江的水流方向为N25~27 E。
海洋动力系统与MASNUM海洋数值模式体系
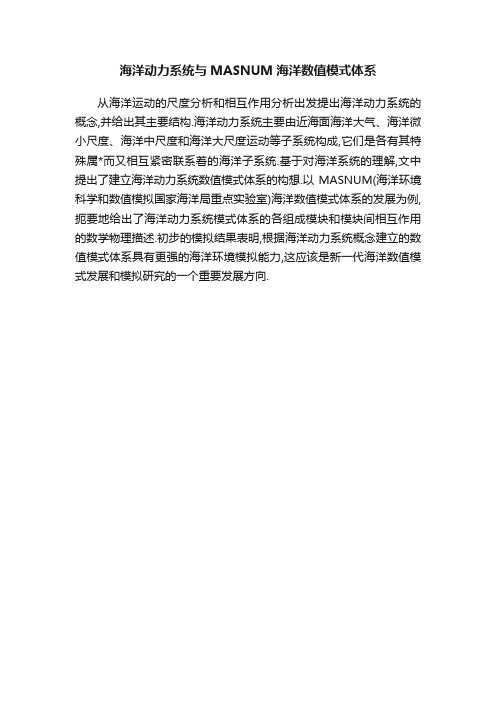
海洋动力系统与MASNUM海洋数值模式体系
从海洋运动的尺度分析和相互作用分析出发提出海洋动力系统的概念,并给出其主要结构.海洋动力系统主要由近海面海洋大气、海洋微小尺度、海洋中尺度和海洋大尺度运动等子系统构成,它们是各有其特殊属*而又相互紧密联系着的海洋子系统.基于对海洋系统的理解,文中提出了建立海洋动力系统数值模式体系的构想.以MASNUM(海洋环境科学和数值模拟国家海洋局重点实验室)海洋数值模式体系的发展为例,扼要地给出了海洋动力系统模式体系的各组成模块和模块间相互作用的数学物理描述.初步的模拟结果表明,根据海洋动力系统概念建立的数值模式体系具有更强的海洋环境模拟能力,这应该是新一代海洋数值模式发展和模拟研究的一个重要发展方向.。
- 1、下载文档前请自行甄别文档内容的完整性,平台不提供额外的编辑、内容补充、找答案等附加服务。
- 2、"仅部分预览"的文档,不可在线预览部分如存在完整性等问题,可反馈申请退款(可完整预览的文档不适用该条件!)。
- 3、如文档侵犯您的权益,请联系客服反馈,我们会尽快为您处理(人工客服工作时间:9:00-18:30)。
Proceedings of Hypothesis IV, 9-14 September 2001, Strahlsund, Germany, p. 368 NUMERICAL SIMULATION OF HYDROGEN RELEASE IN A PRIVATE GARAGEW. Breitung, G. Necker*, B. Kaup, A. Veser*Research Center Karlsruhe, Institute for Nuclear and Energy Technologies, P.O. Box 3640,76021 Karlsruhe, Germany, breitung@iket.fzk.de*ProScience GmbH, Parkstr. 9, 76275 Ettlingen, necker@pro-science.deKey words: Numerical simulation, hydrogen distribution, garage, computational fluid dynamics, risk assessment, safetyAbstract:This paper investigates the formation of combustible clouds from pulsed release of hy-drogen in a garage. The analysis consists of two steps: (1) 3d calculation of the time and space dependent H2-concentration field, and (2) evaluation of the resulting combustion hazard, using newly developed criteria for flame acceleration and detonation potential. In case of a low release rate (0.34 g/s over 100s) the possibility for a slow or fast deflagration exists essentially only during the release period and involves only few g H2. Detonations are excluded. The mixing processes have sufficient time for H2 dilution. In case of the fast release rate (3.4 g/s over 10s) the balance between H2-source and mixing processes is dominated by the source leading to large inhomogeneities, a long term potential for slow deflagration, a short term (20 s) potential for fast turbulent deflagration and detonation. For control of such situations effective mitigation measures appear necessary and feasible.1. Introduction and objectivesWith the introduction of hydrogen fueled passenger cars into the market, the inadvertent release of hydrogen in a private garage will become an important safety issue. The risk of such an event is re-lated to the fact that combustible H2-air clouds will develop in the garage, even when on a homoge-neously mixed basis the average gas composition is far below the flammability limit. The time de-pendent cloud size and its composition result from a complicated interaction between properties of the hydrogen source, the active transport and mixing processes, and the available hydrogen sinks.In this paper we investigate the evolution of combustible H2-air mixtures during H2-release in a garage and evaluate the resulting hazard potential with newly developed criteria. The objective is to quantify the risk for slow and fast combustion modes by calculating associated hazard parameters like size, composition and time of existence of reactive clouds.2. MethodologyA systematic step-by-step procedure for the deterministic analysis of hydrogen behaviour and miti-gation was developed previously in the frame of nuclear safety research programs at FZK [1].The procedure can be subdivided into four main parts (Fig.1):(1) modelling of the H2-air mixture generation within the enclosure,(2) prediction of the hazard potential of this mixture with respect to flammability, flame accel-eration and detonation on-set, using newly developed criteria,(3) numerical simulation of the appropriate combustion regime with verified 3D-computationalfluid dynamics (CFD) codes, and(4) consequence analysis based on the calculated pressure and temperature loads.In this paper we restrict the analysis to the first two parts. Each part consists of several steps. The information needed for each step, and the assumptions made for this analysis are now summarized.2.1 Combustible mixture generationStarting point of any analysis is the geometrical modeling of the building in an appropriate compu-tational grid. The building walls and the inner surfaces define the mathematical boundary conditions for the solution of the 3d fluid-flow equations solved in the CFD model.Fig.1 Step-wise procedure for deterministic analysis of hydrogen behaviour in risk studies.In the present analysis a simple rectangular garage of 2.4 m height, 4.5 m width and 6.5 m length containing a parked vehicle, is modeled. The garage door has two vent openings in the upper re-gion. Otherwise the garage is closed to the outside. Two different meshes were applied in the analy-sis, one consisting of 10´10´10 cm3 cubic mesh cells (» 70 000 cells), the other with a refined mesh of 10´10´3.3 cm3 in the upper 70 cm of the garage volume (» 111 000 cells).For a defined geometry, the next step of the hydrogen analysis is definition of the mitigation meas-ures which are to be included in the modeling. In the present case one could e.g. investigate the ef-fects from additional vent openings, from forced ventilation or from a catalytic garage ceiling. The GASFLOW code includes verified models which allow to predict the effects of these mitigation ap-proaches on the hydrogen distribution process. Mitigation measures are not addressed in this paper due to space limitations.For a given geometry and mitigation system, the next question is what events should be covered in the analysis as representative accident scenarios. For the present study an intermittend release of cold hydrogen gas, which could e.g. represent the boil-off from a cryogenic tank is investigated.After definition of the relevant scenarios, the corresponding hydrogen sources must be specified. For consistent CFD calculations the mass, momentum and energy of the released hydrogen are needed for the whole duration of the simulation. In addition, the location and direction of the H2-source flow must be selected. For estimation of the release rate due to boil-off, a thermal energy deposition into the cryogenic tank of 1 W is assumed. The vaporized liquid (170 g/day) is released in 5 pulses per day (=34 g/pulse) over a period of 10 or 100 seconds, respectively (3.4 and 0.34 g H2/s). The gaseous hydrogen is released at a temperature of 22.3 K underneath the trunk in down-ward direction, which represents conservative assumptions with respect to hydrogen mixing with air. If homogeneously mixed with the available air in the garage, the released H2-mass per pulse (34g) would lead to a concentration far below the flammability limit (0.6% H2). However, due to rate limitations in the physical mixing processes even for such apparently sub-critical H2-sources, heterogeneities and combustible clouds must be expected. The question is where do they develop, how much hydrogen is involved, and what combustion regime could result for what time period. With given characteristics of the hydrogen source, the distribution and mixing with air can be calcu-lated using the verified 3d CFD code GASFLOW [2,3]. The outcome from this step of the analysis are temperature, pressure and composition of the H2-air mixture as function of time and location. In the present case the following physical phenomena must be modeled or considered: single-phase, multicomponent gas flow under the influence of gravity, pressure gradients, viscous stresses and drag from inner surfaces and flow restrictions, molecular transport including hydrogen diffusion, heat transfer from hydrogen (22 K) to air (300 K), and effect of turbulence on mass, energy and momentum transport.2.2 Hazard potentialThe second part of the risk assessment evaluates the hazard potential that the calculated hydrogen-air distribution may represent to structures and persons if an ignition should occur (second column in Fig.1). Within the FZK hydrogen research program three criteria were developed to classify the possible combustion regimes and their consequences. These empirical criteria were derived from available literature data and many own tests with H2-air-mixtures. The criteria capture the observed combustion regimes in a condensed form and allow predictions with respect to the risk of a given theoretically calculated H2-air distribution.We distinguish three hazard levels. The first one is flammability of the mixture. In the present case of pure hydrogen-air mixtures at ambient conditions we assume that flammability exists if either the hydrogen concentration reaches 4 vol % (lower explosion limit) or the oxygen concentration reaches 5 vol% (upper explosion limit, = 75 vol % H2 in air) [4]. To quantify and compare the haz-ard with respect to flammability the burnable volume and hydrogen mass within the range of 4 to 75 vol% H2 was computed for the investigated release scenarios.A second hazard level is reached if the computed mixture can support flame acceleration under conservative conditions, i.e. with high degree of confinement and turbulence generation by flow ob-stacles. The systematic analysis of all experimental data with different scaling methodologies showed that the expansion ratio s (= ratio of specific volumes, m3kg-1, of burned to unburned gas at constant pressure) is the dominating mixture parameter which determines the flame propagation re-gime [5]. The critical s-value above which flame acceleration was observed in H2-air mixtures at ambient initial temperature iss crit = 3.75 ± 0.25. (1)This expansion ratio corresponds to 10.5 vol% H 2 on the lean side, and 75 vol% H 2 on the rich side. For the present analysis the potential for flame acceleration is quantified by computing from the known 3d hydrogen distribution as function of time the cloud volume for which the expansion ratio exceeds the critical value.The third and highest hazard level is reached if the computed H 2-air mixture can support detonation on-set under conservative conditions, i.e. high confinement and turbulence generation. A large number of joint experiments were performed in various facilities of the Kurchatov Institute Moscow and Research Center Karlsruhe [6]. In addition the available literature data on detonation onset con-ditions were collected and critically evaluated [7]. The final result is that a minimum scale require-ment for onset of deflagration-to-detonation-transition (DDT) exists, which can be expressed as: D ³7l (2) where D is the characteristic size of the reactive gas cloud taken as third root of the cloud volume (D=V 1/3) and l is the detonation cell width of the average gas mixture in the reactive cloud. In the present study we have evaluated the ratio D(t)/7l (t) for the flame acceleration cloud (³ 10.5% H 2) during and after the hydrogen release to quantify the potential risk for DDT in the different release scenarios.3. Calculations performed3.1 ScenariosHydrogen is released in a simple rectangular garage with two different rates into initially stagnant air at ambient temperature. Table 1 summarizes the assumed parameters.Table 1 Investigated garage geometry and hydrogen release scenariosGEOMETRY HYDROGEN SOURCE CASE Volume (m 3) Vent Openings H 2-Rate (g/s) Duration (s) Total Mass (g) Release Temp. (K) Release Location Nr. 70.2 Two times 10´20 cm 23.40 10 34 22.3 under- neath 10.34 100 34 22.3 trunk 23.2 GASFLOW sensitivity studyTo investigate the effects from numerical methods on the computed hydrogen distribution in the garage the following modeling variations were made:- first and second order numerical solver (Donor, vanLeer),- equidistant grid (D x=D y=D z=10 cm), and vertically refined grid near the ceiling (D z=3.3 cm), - diffusion terms on and off, k-e turbulence model on and off.The highest correlation was observed between the size of the computed combustible cloud (>4 % H 2) and the used grid resolution. The edge of the cloud is sensitive to numerical diffusion because of its very low H 2-concentration value compared to the source. The inner, more H 2 enriched regions of the combustible cloud, which are evaluated in the s - and l -criterion resp., showed little depend-ence on any of the investigated model variations. The results described in the next section refer to the calculation with second order solver, refined grid, no turbulence and no molecular diffusion. Weconsider this our current best estimate calculation because the neglection of of these processes re-sults in a conservative H2-distribution, and because the isotropic k-e turbulence model is not fully applicable to this special case.4. Results4.1 Hydrogen distributionFig.2 shows typical results for the computed hydrogen distribution in the garage for case 1 (3.4 g H2/s). Initially two separate plumes rise along two sides of the trunk. After reaching the ceil-ing a mushroom shaped cloud forms. About 20 seconds after begin of the release the combustible cloud has obtained its largest extension. The velocities in the rising plume reach about 1 m/s. The momentum in the flow is redirected at the ceiling causing an initially circular expansion of the burnable cloud and then reflections at the nearby walls (Fig.2c). These reflections lead to a prefer-ential flow along the ceiling towards the front of the car (Fig. 2e). Air is entrained by these convec-tive gas motions, leading to decrease of the combustible volume. The calculation finally predicts a stable stratification with most of the released hydrogen in a shallow layer underneath the ceiling.In case of the slow release (0.34 g H2/s) a different distributions process is predicted (Fig.3). Only one thin, tube-like H2-air plume extends from the source below the trunk to the ceiling. The plume pulsates slightly in flow rate and diameter, but is otherwise quasi-stationary. As in case 1, a stable stratified hydrogen distribution develops in the garage, however this time with less gas motion along the ceiling. The stratification existing after the end of the release in the garage is demon-strated in Figures 3d and e. Enough time was available to dilute the released hydrogen practically completely to inert mixtures with less than 4% H2.4.2 Hazard evaluationFor a risk assessment the question must be addressed what the calculated hydrogen distributions in the garage mean in terms of hazard. The three criteria described in Section 3 were therefore evalu-ated from the computed time dependent H2-air distributions. The results for these three hazard lev-els are shown in Fig. 4 for cases 1 and 2.Case 1: The high release rate of 3.4 g H2/s leads to a maximum characteristic size of the combusti-ble cloud near 1.6 m, which is equivalent to 4.1 m3. This maximum value is reached at about 20s. After the end of the release (10s) the combustible cloud still grows due to dilution of its enriched kernel by further entrainment of air. After reaching its maximum the cloud size decreases due to convective mixing by the momentum induced gas flows. Thereafter the volume of the combustible mixture approaches a constant value.The cloud which could support flame acceleration begins to grow directly with the start of the hy-drogen release and reaches a maximum volume of about 0.7 m3 shortly after termination of the re-lease. The corresponding H2 inventory is 20 g. Thereafter the cloud disappears in about 10 seconds. This dynamic growing and shrinking of the sensitive cloud results from the balance between the hydrogen source strength and the mixing processes. In the first phase (< 12s) the release dominates and in the second phase (> 12s) the mixing dominates. Comparison of the time scale shows that the decay of the sensitive cloud (³ 10.5%) feeds the growth of the combustible cloud (³ 4%).The evaluation of the DDT potential shows even higher dynamics. The cloud reaches detonable composition and size about 2s after begin of the hydrogen release. The most sensitive situationa) 7 seconds after begin ofH2 release underneathtrunkb) 10 secondsc) 15 secondsd) 25 secondse) 35 secondsFig. 2: Computed hydrogen concentration fields in the garage, case number 1, isosurface in space for 4 vol% hydrogen, release of 3.4 g H2/s for 10 seconds.a) 20 seconds after beginof release, 4 vol% H2isosurfaceb) 50 seconds, 4 vol% H2c) 80 seconds, 4 vol% H2d) 120 seconds, 2 vol% H2e) 120 seconds, 1 vol% H2Fig. 3: Computed hydrogen concentration fields in the garage, case number 2, isosurfaces in space, release of 0.34 H2/s for 100 seconds.CASE 1CASE 20204060801001201401601800102030405060d (cm)cc d (cm)cc 010203040506070809005010015020025000.10.20.30.40.50.60.70.80102030405060V (m³)fa V (m³)fa 00.020.040.060.080.100.120501001502002500.511.522.530102030DDT no DDT405060D7lno DDT00.20.40.60.81.01.21.4050100150200250D 7lTime (s) Time (s)Fig.4:Computed hazard parameters for Left: Case 1, hydrogen release rate 3.4 g H 2/s for 10 s. Right: Case 2, hydrogen release rate 0.34 g H 2/s for 100 s. Top: Characteristic dimension of the combustible cloud, d cc , with H 2concentrations between 4 and 75 vol% H 2.Middle: Volume of the cloud which could support spontaneous flame acceleration,V fa , volume with H 2-concentrations between 10.5 and 75%.Bottom: DDT index D/7λ of cloud with H 2-concentrations between 10.5 and 75 vol%.exists a few seconds later during which hydrogen accumulates underneath the car. DDT possibility is predicted for a total duration of about 10s (D/7l >1). Analog to above, the decay of the detonable cloud supports the simultaneous growth of the acceleration cloud.Case 2: A different picture evolves for the slow release rate. Fig.4 shows that a much smaller com-bustible cloud is formed in case 2 (d cc £ 0.8 m, V cc £ 0.5 m 3) and that 20s after begin of the H 2 re-lease a quasi-equilibrium is established between the source and the mixing processes, leading to a nearly constant cloud volume (Fig 3b and c). After termination of the source the combustible cloud dissolves within 20 seconds, because the plume is cut off and regions with 4% H 2 or more could not sustain under the ceiling during the long release period.The cloud capable to support flame acceleration is also much smaller in case 2 than in case 1 (about 0.1 m 3 vs. 0.7 m 3). Again a quasi-equilibrium exists between sources and sinks. The cloud disap-pears within 10 s after termination of the hydrogen source. The maximum hydrogen inventory of the flame acceleration cloud was only 2.7 g. The evaluation of the DDT criterion D/7l shows that the size and the hydrogen concentration of the enriched cloud are insufficient for a transition to detonation for practically the whole duration of the hydrogen release.Table 2: Summary of computed hazard parametersCASE FLAMMABLE CLOUD FLAME ACCELERATION CLOUDDETONABLE CLOUDMax.Size (m 3) Time of Existance (s) Max.Size (m 3) Max Mass (gH 2) Time of Exist (s) Max. Size (m 3) Max. Mass(g/H 2)Time ofExist. (s) Case 1 (3.4 gH 2/s) 4.1 complete analysis time (50 s) 0.7 20 release time +15s= 25 s0.7 20 10 Case 2 (0.34g H 2/s) 0.5 release time+15 s= 115 s0.1 2.7 release time +15s = 115 s - - -Table 2 summarizes the computed hazard parameters, which show a strong dependence on the hy-drogen release rate. In case of the low rate only a relatively small flammable cloud develops(<0.5 m 3). Combustible mixtures from during the release period only in the rising plume. Shortly after termination of the source no combustible mixtures are left in the garage and no combustion hazard remains.(Another risk which is not addressed here, would arise if released hydrogen could penetrate into highly confined compartments of the car.)For the large release rate up to about 4 m 3 of combustible mixture develop in the garage. As long as the source is active, an inner kernel of enriched mixtures exists (up to 0.7 m 3) which has the poten-tial for sustaining supersonic combustion modes. These enriched regions dilute however rapidly to more insensitive mixtures after termination of the source. This process of the other hand supports the persistance of the deflagrative cloud (³ 4% H 2), which at the end of the calculation shows a sta-ble stratified distribution near the ceiling of the garage. Accidental ignition of such a mixture would lead to substantial damage of the car and the garage. To control H 2 release rates in the range of grams per second active or passive countermeasures seem necessary, which however need careful design calculations to prove the required efficiency.5. Summary and conclusionsThe risk from pulsed release of hydrogen in a garage was investigated. The analysis concentrated on two examples for “subcritical” hydrogen sources, which when homogeneously distributed in the ga-rage would result in inert mixtures far below the flammability limit (0.6 vol% H2). The presented 3d computations have identified the size, shape, composition and evolution of the reactive H2-air clouds which would develop during and after the pulsed H2-release period. The applied criteria al-lowed to quantify several risk parameters like size, hydrogen mass, and times of existance of the mixtures which under inadvertent conditions could sustain a slow deflagration, a fast turbulent de-flagration or even a detonation (Table 2).The dynamics of the reactive cloud evolution are governed by the balance between the H2 source and the physical mixing mechanisms, which tend to dilute the pure hydrogen released at the H2 exit. In case of the high release rate the source dominated over the mixing processes for the whole re-lease period (10 s), leading to a large and continously growing cloud. In case of the low release rate source and mixing mechanisms reached a quasi-equilibrium, resulting in a small but practically constant cloud size during the release period.After termination of the hydrogen release the sensitive clouds which could support spontaneous flame acceleration (Eq.1) or detonation on-set (Eq.2) disappear for both release rates within 10-15 seconds due to convective mixing. The combustible cloud (³4% H2) behaves differently however. In case of the high release rate a long-term potential for slow deflagration of much of the injected hydrogen exists in form of a stable stratified layer under the ceiling. In case of the low release rate the mixing processes have had sufficient time to prevent the formation of a combustible gas, once the source is terminated.Hydrogen distribution and the associated formation of reactive clouds is a complex process which depends on many parameters. Modern 3d CFD simulations can capture the essential phenomena and make predictions about effective countermeasures for risk control.References[1]W. Breitung, P. Royl, Nuclear Engineering and Design 202 (2000), 249-268[2]J.R. Travis, P. Royl, R. Redlinger, G.A. Necker, J.W. Spore, K.L. Lam, T.L. Wilson,B.D. Nichols,C. Müller, 1998. GASFLOW-II: Theory and Computational Model, Vol 1,Reports FZKA-5994, LA-13357-MS.[3]J.R. Travis, P. Royl, R. Redlinger, G.A. Necker, J.W. Spore, K.L. Lam, T.L. Wilson,B.D. Nichols,C. Müller, 1998. GASFLOW-II: Users Manual, Vol 2, Reports FZKA-5994,LA-13357-MS.[4]U. Bielert, W. Breitung, Proceedings Annual Meeting on Nuclear Technology ‚99, ISSN 0720-9207, Karlsruhe, 18.-20. May 1999, p. 167[5]S.B. Dorofeev, M. Kuznetsov, M. Alekseev, A. Efimenko, A. Bezmelnitsyn, A. Yankin,W. Breitung, Reports IAE-612713, Kurchatov Institute Moscow, Russia, and FZKA6268,Forschungszentrum Karlsruhe, Germany (1999).[6]W. Breitung, S.B. Dorofeev, Proceedings Annual Meeting on Nuclear Technology ’99, ISSN0720-9207, Karlsruhe, Germany 18-20 May 1999, p.185[7]S.B: Dorofeev, Chapter 3 in W. Breitung, C. Chan, S. B. Dorofeev, A. Eder, B. Gelfand, M.Heitsch, R. Klein, A. Malliokos, E. Shepherd, E. Studer, P. Thibault, ReportNEA/CSNI/2000/7 (August 2000), OECD Paris, see also ftp://inrh2b.FZK.de/pub/SOAR.。