Porous silicon formatio A quantum wire effect
超米特电子有限公司产品说明书

1US Headquarters TEL +(1) 781-935-4850FAX +(1) 781-933-4318 • Europe TEL +(44) 1628 404000FAX +(44) 1628 404090Asia Pacific TEL +(852) 2 428 8008FAX +(852) 2 423 8253South America TEL +(55) 11 3917 1099FAX +(55) 11 3917 0817Superior elongation and tensilestrength help to prevent tearing in use due to mishandling. Typical properties for CHO-SEAL 1310 and 1273 materi-al are shown on pages 33 and 32respectively.High Shielding PerformanceCHO-SEAL 1310 material provides more than 80 dB of shielding effectiv-ness from 100 MHz to 10 GHz, while CHO-SEAL 1273 material provides more than 100 dB.Low Volume ResistivityBoth materials have exceptionally low volume resistivity, which makes them well suited for grounding appli-cations in which a flexible electrical contact is needed.Low Compression GasketSpacer gaskets are typicallydesigned to function under low deflec-tion forces. Chomerics uses design tools such as Finite Element Analysis (FEA) to accurately predict compres-sion-deflection behavior of various cross section options. Refer to page16.LCP Plastic SpacerLiquid crystal polymer (LCP)spacers, including those made with Vectra A130 material, provide aCHO-SEAL ®1310 or 1273Conductive ElastomersWith EMI spacer gaskets, shielding and grounding are provided by Chomerics’CHO-SEAL 1310 and 1273 conductive elastomers, specifi-cally formulated for custom shape molded parts. They provide excellent shielding and isolation against electro-magnetic interference (EMI), or act as a low impedance ground path between PCB traces and shielding media. Physically tough, these elas-tomers minimize the risk of gasket damage, in contrast to thin-walled extrusions or unsupported molded gaskets.Silicone-based CHO-SEAL 1310and 1273 materials offer excellent resistance to compression set over a wide temperature range, resulting in years of continuous service. CHO-SEAL 1310 material is filled with silver-plated-glass particles, while 1273 utilizes silver-plated-copper filler to provide higher levels of EMI shielding effectiveness.EMI Spacer GasketsThe unique design of Chomerics’EMI spacer gaskets features a thin plastic retainer frame onto which a conductive elastomer is molded. The elastomer can be located inside or outside the retainer frame, as well as on its top and bottom surface. EMI spacer gaskets provide a newapproach to designing EMI gaskets into handheld electronics such as dig-ital cellular phones. Board-to-board spacing is custom designed to fit broad application needs. Customized cross sections and spacer shapes allow for very low closure forcerequirements and a perfect fit in any design or device.Robotic InstallationSpacer gaskets can be installed quickly by robotic application. Integral locater pins in the plastic spacer help ensure accuratepositioning in both manual and pick-and-place assembly. Benefits include faster assembly and lower labor costs.The integrated conductive elastomer/plastic spacer gasket is a low cost,easily installed system for providing EMI shielding and grounding in small electronic enclosures.Figure 1Single Piece EMI Gasket/Locator PinsCHO-SEAL 1310 or 1273 Conductive Elastomer (Inside)Plastic Spacer Around Outsideor InsideApplications for EMI Spacer GasketsThe spacer gasket concept is especially suited to digital and dual board telephone handsets or other handheld electronic devices. It provides a low impedance path between peripheral ground traces on printed circuit boards and components such as:•the conductive coating on a plastic housing•another printed circuit board •the keypad assemblyTypical applications for EMI spacer gaskets include:•Digital cellular, handyphone and personal communications services (PCS) handsets •PCMCIA cards•Global Positioning Systems (GPS)•Radio receivers•Other handheld electronics, e.g.,personal digital assistants (PDAs)•Replacements for metal EMI shield-ing “fences” on printedcircuit boards in wireless tele-communications devicesstable platform for direct, highprecision molding of conductive elas-tomers. The Vectra A130 material described in Table 1 has excellent heat deflection temperature character-istics (489°F, 254°C). For weight con-siderations, the LCP has aspecific gravity of only 1.61. This plas-tic is also 100% recyclable.Typical EMI Spacer Gasket Design ParametersThe EMI spacer gasket concept can be considered using the design parameters shown in Table 2. Some typical spacer gasket profiles are shown below.Figure 2Typical Spacer Gasket Profiles3US Headquarters TEL +(1) 781-935-4850FAX +(1) 781-933-4318 • Europe TEL +(44) 1628 404000FAX +(44) 1628 404090Asia Pacific TEL +(852) 2 428 8008FAX +(852) 2 423 8253South America TEL +(55) 11 3917 1099FAX +(55) 11 3917 0817Finite Element AnalysisChomerics, a division of the Parker Hannifin Corporation’s Seal Group, is the headquarters of Parker Seal’s Elastomer Simulation Group. This unit specializes in elastomer finite element analysis (FEA) using MARC K6 series software as a foundation for FEA capability.Benefits of FEA include:•Quickly optimizing elastomer gasket designs•Allowing accurate predictions of alternate elastomer design concepts •Eliminating extensive trial and error prototype evaluationTypical use of FEA in EMI spacer gasket designs is to evaluate the force vs. deflection requirements of alternate designs.For example, onespacer design features a continuous bead of con-ductive elastomer molded onto a plastic spacer. An alternative designemploys an “interrupted bead,” where the interrup-tions (gaps left on the plastic frame) are sized to maintain the requiredlevel of EMI shielding. Figure 4illustrates these alternative designs.Gasket DeflectionFigure 5 compares the effect of continuous and interrupted elastomer gasket designs in terms of the force required to deflect the conductive elastomer. This actual cellular handset application required a spacer gasket with interrupted bead to meet desired deflection forces.Chomerics Designand Application ServicesChomerics will custom design a spacer for your application. Advice,analysis and design assistance will be provided by Chomerics Applications and Design engineers at no additional fee. Contact Chomerics directlyat the locations listed at the bottom of the page.Figure 3FEA Example of an EMISpacer Gasket Cross SectionFigure 4Continuous (top) and InterruptedElastomer GasketsFigure 5Typical Spacer Gasket Deflection。
自粘型聚硼硅氧烷复合材料性能
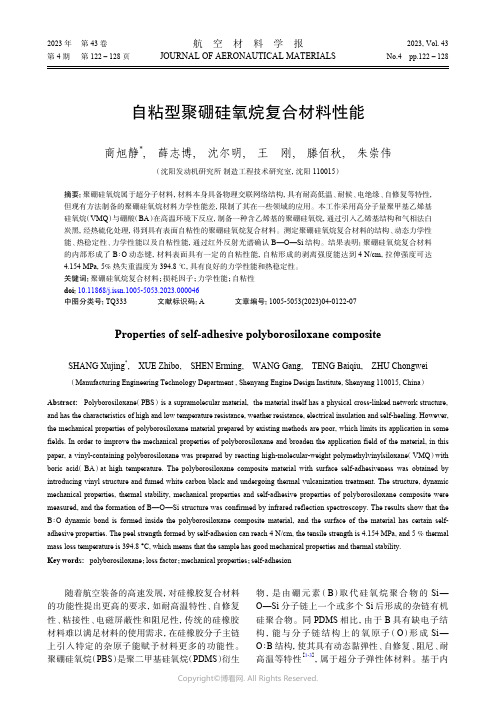
自粘型聚硼硅氧烷复合材料性能商旭静*, 薛志博, 沈尔明, 王 刚, 滕佰秋, 朱崇伟(沈阳发动机研究所 制造工程技术研究室,沈阳 110015)摘要:聚硼硅氧烷属于超分子材料,材料本身具备物理交联网络结构,具有耐高低温、耐候、电绝缘、自修复等特性,但现有方法制备的聚硼硅氧烷材料力学性能差,限制了其在一些领域的应用。
本工作采用高分子量聚甲基乙烯基硅氧烷(VMQ)与硼酸(BA)在高温环境下反应,制备一种含乙烯基的聚硼硅氧烷,通过引入乙烯基结构和气相法白炭黑,经热硫化处理,得到具有表面自粘性的聚硼硅氧烷复合材料。
测定聚硼硅氧烷复合材料的结构、动态力学性能、热稳定性、力学性能以及自粘性能,通过红外反射光谱确认B—O—Si结构。
结果表明:聚硼硅氧烷复合材料的内部形成了B∶O动态键,材料表面具有一定的自粘性能,自粘形成的剥离强度能达到4 N/cm,拉伸强度可达4.154 MPa,5%热失重温度为394.8 ℃,具有良好的力学性能和热稳定性。
关键词:聚硼硅氧烷复合材料;损耗因子;力学性能;自粘性doi:10.11868/j.issn.1005-5053.2023.000046中图分类号:TQ333 文献标识码:A 文章编号:1005-5053(2023)04-0122-07Properties of self-adhesive polyborosiloxane compositeSHANG Xujing*, XUE Zhibo, SHEN Erming, WANG Gang, TENG Baiqiu, ZHU Chongwei(Manufacturing Engineering Technology Department , Shenyang Engine Design Institute, Shenyang 110015, China)Abstract: Polyborosiloxane(PBS) is a supramolecular material, the material itself has a physical cross-linked network structure, and has the characteristics of high and low temperature resistance, weather resistance, electrical insulation and self-healing. However, the mechanical properties of polyborosiloxane material prepared by existing methods are poor, which limits its application in some fields. In order to improve the mechanical properties of polyborosiloxane and broaden the application field of the material, in this paper, a vinyl-containing polyborosiloxane was prepared by reacting high-molecular-weight polymethylvinylsiloxane(VMQ)with boric acid(BA)at high temperature. The polyborosiloxane composite material with surface self-adhesiveness was obtained by introducing vinyl structure and fumed white carbon black and undergoing thermal vulcanization treatment. The structure, dynamic mechanical properties, thermal stability, mechanical properties and self-adhesive properties of polyborosiloxane composite were measured, and the formation of B—O—Si structure was confirmed by infrared reflection spectroscopy. The results show that the B∶O dynamic bond is formed inside the polyborosiloxane composite material, and the surface of the material has certain self-adhesive properties. The peel strength formed by self-adhesion can reach 4 N/cm, the tensile strength is 4.154 MPa, and 5 % thermal mass loss temperature is 394.8 °C, which means that the sample has good mechanical properties and thermal stability.Key words: polyborosiloxane;loss factor;mechanical properties;self-adhesion随着航空装备的高速发展,对硅橡胶复合材料的功能性提出更高的要求,如耐高温特性、自修复性、粘接性、电磁屏蔽性和阻尼性,传统的硅橡胶材料难以满足材料的使用需求,在硅橡胶分子主链上引入特定的杂原子能赋予材料更多的功能性。
多孔硅激光英文
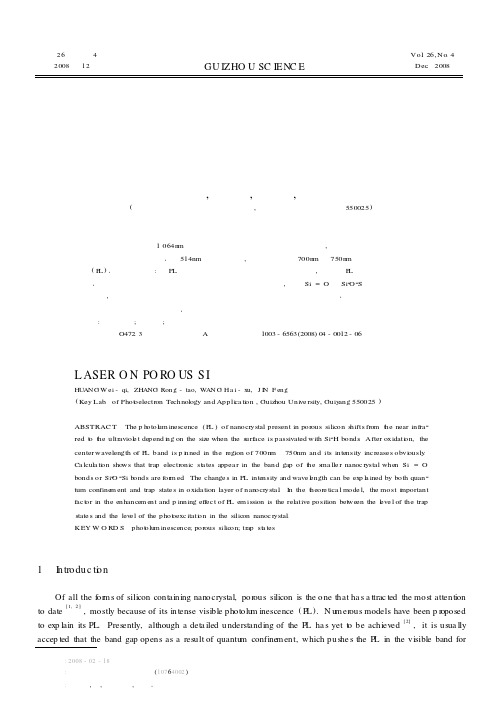
第26卷 第4期2008年12月 贵 州 科 学GU IZHO U SC IENC E Vol.26,No.4Dec.2008收稿日期资助项目国家自然科学基金资助项目(6)作者简介黄伟其,男,贵州大学,教授1多孔硅激光黄伟其,张荣涛,王海旭,金 锋(贵州光电子技术与应用重点实验室,贵州大学 贵州 贵阳 550025)摘 要 我们用波长为1064n m的纳秒脉冲激光在硅表面打出小孔结构,然后再做高温退火处理形成了硅的纳米氧化低维结构1在514nm激光泵浦下,发现该结构在700n m和750n m处有很强的受激辐射发光(P L)1实验中发现:该PL发光有明显的阈值表现和激光增强效应,证明该P L发光确实是光致受激发射1计算給出氧化界面态模型来解释该光致受激发光机理,其中Si=O和Si2O2S的键合可以产生氧化陷阱态,关键在于该氧化陷阱态与价带顶空穴态之间能够形成粒子数反转1为硅基上激光器件的光电子集成研发开辟了新的途经1关键词:光致荧光;多孔硅;陷阱态中图分类号 O472.3 文献标识码 A 文章编号 1003-6563(2008)04-0012-06L ASER O N PO RO US S IHUAN G W ei-qi,ZHANG Rong-tao,WAN G H a i-x u,J I N F eng(Key Lab.of Phot oelectron Technology and Appli ca ti on,Guizhou Uni ve rsity,Guiy ang550025)ABSTRAC T The p h otol um inescence(P L)of nanocrystal present in porous silicon s hifts fro m t he near infra2red t o t he ultraviol e t depend i ng on the size when the s urface is passivated with Si2H bonds.After oxidati on,thecenter wavelength of P L band is p i nned in the region of700n m~750nm and its intensity inc rease s obvi ously.Ca lcula ti on sho ws that trap electronic st a tes appea r in the band gap of t he s ma lle r nanoc rystal when Si=Obonds or Si2O2Si b onds are for m ed.The change s in PL intensity and wave l ength can be ex p l a ined by both quan2tum confine m ent and trap state s in oxida tion layer of nan ocrysta l.In the t heore tica l mode l,the most i m portantfac t or in the enhancem ent and p inning effec t of P L em ission is the relative position bet ween the l eve l of the trapstate s and the lev e l of the phot oexc itati on in the silicon nanoc rystal.KEY W O RD S phot olu m inescence;porous silicon;trap st a tes1 I ntroduc ti o nOf all the f or m s of silicon containing nanocrystal,por ous silicon is the one that ha s a ttrac ted the most attention to date[1,2],mostly because of its intense visible photolu m inescence(P L).N u m er ous models have been p r oposed to exp lain its PL.Presently,although a deta iled understanding of the P L ha s yet t o be achieved[2],it is usua lly accep ted that the band gap opens as a result of quantu m confine m ent,which pushe s the P L in the visible band for:2008-02-18:1074002:nanocrystal .P L of nanocrysta l present in por ous silicon can be turned fr om the nea r infrared t o the ultravi olet when the surf ace is passiva ted with Si 2H bonds .After oxida tion,the centerwavelength of P L band isp inned in the r egion of 700nm ~750nm.M.V .W olkin pr opose a model f or exp laining the p inning wavelength eff ect which is r e lated to the trapp ing of an electr on by Si =O bonds tha t p r oduce localized levels in the bandgap of s m aller nanocrystals[3].I n our calculation the Si 2O 2Si bonds or Si =O bonds on the surface of nanocrystal can all pr oduce the tr ap states .I nW olkin ’s model,it is indicated tha t the quantum confine m ent alone can exp lain the P L on por ous nano 2c r ystals lar ger than 3nm (displaying red or orange e m issi on),and the oxidati on of the nanocrystal p lays an i mpor 2tant r ole as the nanoc r ystal size s ar e s m aller than 3nm.B ut it is different in Q in ’s model that t w o c ompetitive pr ocesse s take place in P L in which the oxidati on state s play a m ain r ole as the size of nanocrystal isla r ger than 3nm ,and the quantum confine m ent take s a dom inant affecti on as the size of nanoc r ystal is s m aller than 3nm [4].The 3nm is a c ritica l size in their models .W e think that the 3nm size is not i mportant and the r e la 2tive positi on bet ween the level of the tr ap states and the level of the phot oexcitati on in the silicon nanoc r ysta l is the most i m portant thing in our model .2 Exp e ri m e nta l p rocedureThe sample s used in the study were p repa r ed as f oll ow ing (A )and (B ),respectively .(A ).W e took som e Si of P 2type (100)orientedwafersw ith a 10220Ωc m as the silicon sa mp les .Having been c leaned in a Summ a cleaner (a m ixture of choline and m ethanol ),the silic on sample swer e prepared by anodizingin HF (49%):Ethanol (1∶1.5)for 15m in.Anodizing current density was about 100mA /cm 2.(B ).The silicon sa mp les were p r epared by anodizing in HF (49%):Ethanol (1∶1.5)f or 15m in unde r the ir 2radia tion of a la ser bea m (spot dia m eter :700μm )with 532nm wave length and 20mW powe r .A nodizing current density was about 60mA /c m 2.After etching,a ll sa mp les we r e rinsed in ethanol and then exposed t o air f or seve r al days .Scanning e lec tr on m icr oscopy (EP MA 21600)was used to obse r ve the oxidati on por ous structures on the sam 2ple s .The P L s pectr a of the sa mp les under the 514nm excita tion we r e m ea sur ed by using REN I S HA W R a man Sys 2te m s in which the spot dia m eter of lase r bea m f or exciting can reach t o 1μm.F ig .1(a )and (b )show the i mages of SE M on the sample s (A )and (B ),r e s pective ly .And their P L spectra a r e co mpa r ed in Fig .1(c ).Fig .1(b)shows a kind of condensed r ods structure in the r egion with irradia ting and anodizing on the sa mpleB.It is c lea r that the laser irradiati on makes the por ous layer c r ack,sc r e w and condensate into s om e r ods state .It is the r od that e m itsmore P L than that of the general por ous sa mp les as shown in Fig .1(c )because of increasing surface on the r ods lar gely and inc r easing por osities .3 C a lcula ti o n m e thodF or num erical calculations,we start fr om a supercell built by j oining f our cubic c 2Si cells on the x 2y plane,and then square e mp ty colum ns are dug a l ong the z 2direc tion by r emoving 13centre Si a t om s [5],which pr oduce a weight por osity of about 40%.The sur face dangling bonds are sa tur a ted by using hydr ogen and oxygen at om s,in or der to si m ulate different degrees of sa m ple oxidati on .This pore mode l based on supercells has the advantage of being si mple and e mphasize s the quantum confine m ent ef fect and def or m ati on of the str uc ture .The r e is pref e r entialf [1]y 2y ()22S f S 2T y 2q 2y T DFT y y x 31 4期 黄伟其,等:多孔硅激光gr owth o por e s al ong the c r stall ogr aphic directi ons in p t pe 001oriented c i wa ers .o we chose the a bove model .he e lec tronic behavi our is inve stigated in the work b an ab initio non relativistic uantum m echani cal anal sis .he calculati on were carried out b using the local densit appr o i m ation41贵 州 科 学 26卷 51 4期 黄伟其,等:多孔硅激光F i g .5 (a )A sha rp d i s tribu ti on m ode l fo r trap s ta te s by contro l ling oxida ti on on po r o usS i i n wh i ch the popu l a ti o n i nve rs i o n o cc ur p roba bly;(b )La se r on P o r o us S i (LDA )and nonlocal gradient 2correc ted exchange 2correla tion f uncti ona l (GG A )f or the self 2c onsistent t otal en 2e r gy calculati on .It is conside r ed tha t both LDA and GG A unde r esti m ate the band gap by 30%250%for sem icon 2duct ors and insulators .The ab initio calculati on in the study have been pe r f or med with the CASTEP code s deve l oped at Ca m bridge U 2niversity,UK,which is considered a s one of the p recise DFT plane 2wave pseudopotential p r ogra m s .The pore sur face is initially saturated by hydr ogen atom s,and during the oxida tion p r ocess they are gr adua lly substituted by oxygen at om s as shown in F ig .2and F ig .3.4 R e su lts and dis cus s i o nW hen nanoc r ystalline Si is oxidized,the Si 2Si or Si 2O 2Si bonds a r e likely to weaken or break because of the la r ge str e ss a t the distorti on surface a s shown in Fig .2(a)and (d)which involves thr ee unsaturated Si 2O 2bonds .Then the Si =O double bond is more likely to be for m ed and stabilize the interface,since it requires neither a la r ge def or m ation ene r gy nor an exce ss e le m ent,while the l ocalized state s are f or m ed in gap obvi ously .Fig .2(b )and (e )show the structure and the density of state s (DOS )in the por e supercell sa tur a ted by t wo Si =O bonds (r e sting one unsaturated Si 2O 2bond ).Fig .2(c )and (f )sho w the str uc tur e and the density of states (DOS )in the pore supe r cell satur a ted by three Si =O bonds (the other dangling bonds be ing satur a ted by hydr ogen a t om s ).On the other hand,the Si 2O 2Si bonds f or m ed on nanocrystalline Si can als o p r oduce the l ocalized states in gap a s shown in F ig .3.Accor ding to the Fourier transf or m infrar ed spec trosc opy (FTI R )[3],the oxide laye r on por ous Si nanocrystal involves the Si =O bonds and the Si 2O 2Si bonds .I n our model,if the Si nanocrystallite is passivated by oxygen,the l ocalized tra p states of electr ons (or even the trapped exciton )may be f or med on the Si =O bonds or Si 2O 2Si bonds .As shown in F ig .4,the model includes t wo syste m s:the left one is a tra p state syste m in the oxidati on layer in which the Si =O bonds and the Si 2O 2Si bonds de ter m ine the trapped electr on state energy which is size inde 2pendent,and the right one is a phot on pump syste m in the Si nanocrystal in which the leve l of the valence band val 2ley depends on nanocrysta l size by quantu m c onfine ment .The position of the tr ap sta tes is in the r egion of 1.5~2.1e V after considering the underesti ma tion of the band gap by the LDA and G G A ca lculation .I ,z f 3,f f L f f f x y f xy y F ()ff f L 61贵 州 科 学 26卷 n the model the critical si e o nm is not i mportant and it is the most i mportant ac t or or P p inning and enhancing that the leve l position o the trap states is l ower than tha t o the electr onic state s o the phot oe citati on in the nanoc r sta l a te r o gen passivit.ig .4a shows the obvious e ects o the P pinning and enhanc ing inwhich the elec tr ons fr om the va lley of the conductor band opened by quantum confine m ent are ra p idly caught into the trap states in gap,then the population inversi on bet ween the top of the valence band and the trap states is thuspossible due to the longer lifeti m e of the tr apped e lec tr ons [3].B ut as shown in F ig .4(b ),the PL p inning and en 2hancing eff ects disappear at all when the level positi on of the trap states is higher than that of the electr onic states of the photoexcita tion in the nanocr ystal .The process in F ig .4(c )is a conversion situa tion of PL pinning and enhan 2c ing in which only a botto m party of oxide states can capture the e lec tr ons in conductor band .After oxida tion,the energy of the oxide sta tes increase s slowly with deceasing of the nanocrystal siz e because of dist orti on which is different fr om the quantum confine m ent [3].For example,the 5n m nanocrystal after oxygen pas 2sivity relate s to about 1.5e V in the energy of the oxide states,and the s m aller nanoc r ysta l (<2nm )t o 2.1e V.The results can exp lain the P L p inning and enhancing effec t in s m aller nanocrystals and the little shift of PL wave length in larger nanocrystals .I n Fig .1(c ),the r ange of the P L band on the nanocrystal passivated with oxygen is fr om 600n m t o 830n m which sho w s that the agreement bet ween experi m ents and the ory is good .W e could make the trap states distribute t o a shar p regi on by contr olling oxidation in irradiation and annea ling a s shown in F ig .5(a)whose laser with three 2level or f our 2level syste m has been realized.Fig .5(b)shows the shar p em issi on sti m ulated in the lase r on por ous Si .5 Conc l u sionI n summ ary,the results in calculati on and expe ri m ent in the paper indicate tha t :(1)W hen the nanocrystal 2line Si is oxidized,the Si 2O 2Si bonds or Si =O bonds can pr oduce the trap states in gap;(2)W hen the band gap of Si nanocrystals opened by quantum c onfinement is w ider than the ga p bet ween the trap state and the valence band,the r ecombinati on of the electr on trapped in oxygen 2related loca liz ed sta tes and the hole on the va lence band (or even trapped exc iton )gene r a tes the P L p inning and enhancing effects;(3)The wavelength distributi on in P L band should be due t o the energy distributi on of the trap sta tes because the P L em issi on co m es fr om the jumping down of the electr ons in the tr ap states;(4)The shar p distributi on of the trap state s f or med by c ontr olling oxida tion in irradia tion and annealing could p r oduce a laser on por ous Si .A cknow ledg m en tThiswork wa s supported by the N ati ona l Na tur a l Science Founda tion of China .R e fe rence s[1] W.Q .Huang,S .R.Liu 1and Fen J i n 1Sti m ulated em issi on fro m trap e lectroni c state s in oxide of nan ocrysta l Si[J ]1Appl .Phy s .L ett .2008,92,2219101[2] S .G .Pavl ov,H .W.Hubers,J .N.Hovenier,Silicon donor and st okes terahertz lase rs[J ]1J.L u m in .2006,121,3041[3] M.V .Wol kin,J.Jorne,P .M.Fauche t,G .A llan,and C.Delerue 1Electr onic sta tes and l um i nescence i n porous silicon quantu mdots :the role of ox ygen[J ]1P hys .Rev .L ett .1999,82,197-2501[4] G .G .Q i n and G .Q in 1M ulti p le mechanis m mode l for phot olu m ine scence fro m oxidi zed por ous Si [J ]1P hys .Sta tus .Solid A ,2000,182,335-3391[5] W Q ,S R L 1L X 1S f x f y S []1y ,,53571 4期 黄伟其,等:多孔硅激光..H uang ..iu and i u ti m u l a ted em issi on r om trap e l ec tron ic state s i n o ide o nano cr stal i J J.App l .P h s .2007102017。
Study of porous glass doped with quantum dots or laser dyes under alpha irradiation
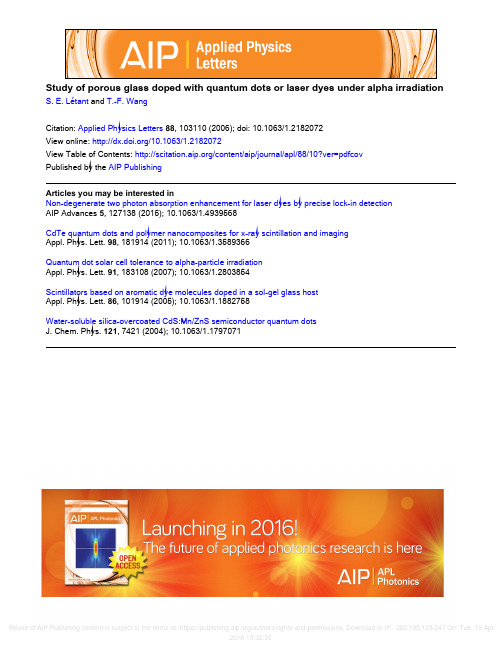
Study of porous glass doped with quantum dots or laser dyes under alpha irradiationS. E. Létant and T.-F. WangCitation: Applied Physics Letters 88, 103110 (2006); doi: 10.1063/1.2182072View online: /10.1063/1.2182072View Table of Contents: /content/aip/journal/apl/88/10?ver=pdfcovPublished by the AIP PublishingArticles you may be interested inNon-degenerate two photon absorption enhancement for laser dyes by precise lock-in detectionAIP Advances 5, 127138 (2015); 10.1063/1.4939568CdTe quantum dots and polymer nanocomposites for x-ray scintillation and imagingAppl. Phys. Lett. 98, 181914 (2011); 10.1063/1.3589366Quantum dot solar cell tolerance to alpha-particle irradiationAppl. Phys. Lett. 91, 183108 (2007); 10.1063/1.2803854Scintillators based on aromatic dye molecules doped in a sol-gel glass hostAppl. Phys. Lett. 86, 101914 (2005); 10.1063/1.1882758Water-soluble silica-overcoated CdS:Mn/ZnS semiconductor quantum dotsJ. Chem. Phys. 121, 7421 (2004); 10.1063/1.1797071Reuse of AIP Publishing content is subject to the terms at: https:///authors/rights-and-permissions. Download to IP: 202.195.129.247 On: Tue, 19 Apr2016 13:32:35Study of porous glass doped with quantum dots or laser dyes under alpha irradiationS.E.Létant and T.-F.Wang a ͒Chemistry and Materials Science Directorate,Lawrence Livermore National Laboratory,7000East Avenue,Livermore,California 94550͑Received 20June 2005;accepted 24January 2006;published online 8March 2006͒We demonstrate that nanocomposite materials based on semiconductor quantum dots have potential for radiation detection via scintillation.While quantum dots and laser dyes both emit in the visible range at room temperature,the Stokes shift of the dyes is significantly larger.The scintillation output of both systems was studied under alpha irradiation and interpreted using a combination of energy loss and photon transport Monte Carlo simulation models.The comparison of the two systems,which allows the quantification of the role played by the Stokes shift in the scintillation output,opens up exciting possibilities for a new class of scintillators that would take advantage of the limitless assembly of nanocrystals in large,transparent,and sturdy matrices.©2006American Institute of Physics .͓DOI:10.1063/1.2182072͔Although both the synthesis and characterization of quantum dots have been developed for more than a decade,1,2the applications explored to date seem to have focused on tagging,3chemical and biological sensing,4and lasing applications.5The interest generated by quantum dots in both academic and industrial research communities comes from the fact that the optical properties of these materials are di-rectly tied to their composition,size,and geometry,therefore allowing the engineering of key parameters,such as emission wavelength and quantum efficiency.Standard gamma-ray detection technology relies on cooled germanium detectors ͑0.2%energy resolution at 1.33MeV ͒6–8and on scintillating crystals such as sodium iodide ͑7%energy resolution at 662keV ͒.9The main prob-lem associated with the former is the necessity to cool and stabilize the detector at a temperature near liquid nitrogen to reduce thermal noise.The main problem associated with the latter is its poor energy resolution.The use of nanocomposite materials could potentially lead to a new class of scintillators that would operate at room temperature,and more importantly,that would not rely on crystal growth,but on the assembly of nanometer-sized crys-tals in a transparent and sturdy matrix.Moreover,scintillator materials have output wavelengths in the ultraviolet ͑UV ͒and blue ͑the most commonly used scintillator,sodium io-dide,emits at 460nm ͒,wavelengths at which the quantum efficiency of photomultiplier tubes ͑PMTs ͒is below 25%.10This means that,even in an ideal situation,only 14of the photons produced in the scintillator material are detected.The use of quantum dots as a scintillator medium would allow fine tuning of the output wavelength in the visible range and therefore,the use of avalanche photodiodes ͑APD ͒with quantum efficiencies up to 70%.11The visible band gap of quantum dots would ensure both high photon output and efficient photon counting.Only one preliminary example of a nanocrystal-based scintillator has been reported to date.12In this work,core/shell ͑CdSe ͒ZnS quantum dots were embedded into a thinlithiated sol-gel matrix,characterized optically,and tested with an alpha source.Unfortunately,no conclusion can be drawn from this publication since the only scintillation spec-trum presented was not corrected from the background radia-tion.In this letter,we demonstrate the ability of quantum dots to convert alpha radiation into visible photons,compare the scintillation output of quantum dots and laser dye mol-ecules,and interpret the results using a combination of energy-loss and photon transport models.This set of data allows us to discuss the limitations of basic quantum dots for gamma-radiation detection applications that require large volumes of scintillator material,and to suggest a new route to solve these issues.Porous VYCOR®was purchased from Advanced Glass and Ceramics ͑Holden,MA ͒in 1/16in.thick sheets.As re-ceived,the material is constituted of an array of intercon-nected pores with a diameter of 4nm and is opalescent.The porous glass matrix was slowly dissolved for 4days in an aqueous solution containing 1%of hydrofluoric acid and 20%of ethanol per volume,rinsed in ethanol,and dried in air.The purpose of this step was to slightly enhance the pore size and to obtain a clear matrix.Scanning electron micro-scope ͑SEM ͒top views of the material recorded after etch-ing,cleaning,and drying without applying any conductive coating on the sample surface ͓see Fig.1͑a ͔͒revealed an average pore diameter in the 10–20nm range.The absorp-tion curve of the same sample shows very good transparency in the visible range ͓see Fig.1͑b ͔͒.Porous glass constitutes a test matrix of choice for scintillation applications because it is made of a succession of nanometer-sized cavities that can hold guest molecules while separating them from each other,therefore preventing self-quenching effects.In addition,it is sturdy,inert,and transparent.CdSe/ZnS core-shell quantum dots with a luminescence output at 540nm were purchased from Evident Technologies ͑Troy,NY ͒and suspended in toluene at a concentration of 10mg/mL.Rhodamine B laser dye was purchased from Sigma-Aldrich ͑Milwaukee,WI ͒and suspended in methanol at a concentration of 0.5mM.The dry “thirsty”porous glass samples were immersed in the solutions of dots or dyes for 48h with continuous stirring in order to allow homogeneousa ͒Author to whom correspondence should be addressed;electronic mail:wang6@APPLIED PHYSICS LETTERS 88,103110͑2006͒0003-6951/2006/88͑10͒/103110/3/$23.00©2006American Institute of Physics88,103110-1 Reuse of AIP Publishing content is subject to the terms at: https:///authors/rights-and-permissions. Download to IP: 202.195.129.247 On: Tue, 19 Apr2016 13:32:35diffusion of the guest molecules into the nanoporous hostmatrix.They were then let to dry in order to evaporate the solvent.Absorbance spectra of the samples were recorded using a UV-visible spectrometer ͑Cary 100,Varian Inc.͒and emis-sion spectra were recorded using a fluorimeter fitted with an optic fiber ͑Cary Eclipse,Varian Inc.͒.The spectra,as well as pictures of the devices,are presented in Fig.2.Although both quantum dots and laser dyes present similarities,such as their tunable absorption and emission spectra in the visiblerange,one noticeable difference is that laser dye molecules have an inherent Stokes shift ͑shift between absorption and emission spectra ͒due to vibrational energy levels,while quantum dots do not.An alpha source ͑243–244Cm,0.2C ͒was placed on one side of the 1/16in.thick nanocomposite sample and a PMT ͑model R1924A from Hamamatsu ͒probing a 1.5cm diam-eter area rested directly on the other side to measure the scintillation output.The PMT output was amplified,digitized by the multichannel analyzer and collected for 10h in order to obtain a histogram.Figure 3shows the scintillation histo-gram recorded with the 243–244Cm source,through the dot and dye samples.The histograms are plotted in counts per channel,where each channel represents the scintillation en-ergy digitized by the multichannel analyzer.The data pre-sented in Fig.3shows that the quantum dot system does convert the alpha-radiation into visible photons,but that the scintillation output of the laser dye system is significantly higher,showing partial energy resolution of the 243–244Cm source.In order to specifically understand the role played by the Stokes shift on the energy resolution for both dye and dot systems,as well as to account for the wavelength mismatch between the emission of the sample and the optimal detec-tion of the PMT,we performed simulations using a combi-nation of TRIM and DETECT 2000programs in history mode.13,14Photons generated at the source/sample interface traveled to the PMT surface which was located on the oppo-site side of the sample.The history was analyzed and the energy deposition and light transport were simulated while accounting for properties of the scintillator media such as the wavelength-dependent absorption and emission coefficients of the emitters,the nature of the matrix ͑reflectivity,indexof FIG.1.͑Color ͒Characterization of the etched,cleaned,and dried porous glass matrix:͑a ͒SEM top views,without any conductive coating.͑b ͒Ab-sorbance spectrum of a 1/16in.thickslab.FIG.2.͑Color ͒Absorbance and emission spectra of ͑a ͒porous glass im-pregnated with quantum dots,and ͑b ͒porous glass impregnated with Rhodamine B laser dye.Pictures of the devices are presented in theinset.FIG.3.͑Color ͒Background-subtracted PMT output ͑energy response ͒upon exposure to a 243–244Cm source located 1mm away from the surface,for ͑a ͒porous glass impregnated with quantum dots,and ͑b ͒porous glass impreg-nated with Rhodamine B dye.The peak recorded for the dye sample was fitted with a Gaussian curve ͑black line ͒.The spectrum of the alpha source recorded by a Si ͑SB ͒detector in the ideal case where both source and detector are in a vacuum is presented in the inset for reference.Reuse of AIP Publishing content is subject to the terms at: https:///authors/rights-and-permissions. Download to IP: 202.195.129.247 On: Tue, 19 Apr 2016 13:32:35refraction,scattering length,and absorption length͒,and thescintillation decay times.The wavelength-dependent quantum efficiency of thePMT was entered into the simulation code.We found that inour unoptimized experimental conditions,4.1%of the pho-tons generated in the dye system were amplified by the PMT,and that0.4%were amplified for the dot system.Replacingthe PMT,which only has a quantum efficiency of4%in thegreen,by an APD,which has a maximum quantum efficiencyof70%,would lead to an improvement of these values by anorder of magnitude͑38%of the photons amplified for thedye system and3.6%for the dot system͒.Many other factors contribute to the observed energyresolution,15such as the intrinsic properties of the scintillatorand the transport efficiency of the photons.For an idealscintillator,the energy resolution R,is given by R=͑⌬E/E͒FWHM=2.35͓͑1+v͑M͒͒/͑Np͔͒1/2,where N is the average number of photons generated at a given energy E, v͑M͒is the variance in the multiplication factor of the PMT, and p is the average transport efficiency.15For a typical10stage PMT͑Ref.16͒with a gain of2.106,v͑M͒is approxi-mately0.08.For the dye system,wefitted the experimentalpeak with a Gaussian͓see Fig.3͑b͒,inset͔and obtained anenergy resolution R of0.64.The p value calculated with DETECT2000being0.041,an estimation of the number of photons N generated at5MeV in the dye nanocomposite is400.For the dot nanocomposite,no⌬E/E can be determinedexperimentally since no peak was detected.This result is inagreement with the simulations,which indicate that if anequivalent photon number N is inputted in the formula tocalculate R,no energy resolution is expected.The compari-son of the dot and dye systems constitutes an important steptoward the understanding of the role played by the spectralproperties of the dye and dot emitters in the scintillationoutput of these nanocomposite systems.After inserting thewavelength-dependent PMT efficiency,and the lifetimes,theonly major difference left between the two systems is theStokes shift,which is significantly smaller for the dots.Wetherefore attribute the output difference of these two systemsto the Stokes shift,which governs the photon transport effi-ciency in the material.Although the number of photons generated in the presentnanocomposite systems is low,this number can change dra-matically for thicker samples studied with penetrablegamma-rays.Moreover,the present systems have neitherbeen optimized for loss minimization͑reflective coating onall surfaces͒nor for detection efficiency͑APD instead ofPMT͒.Ultimately,simulations show that an energy resolutionof2%could be achieved for quantum dot nanocompositematerials due to their visible band gap assuming that the system is linear.This approach would provide gamma-ray detectors with an energy resolution between the cooled semi-conductor detectors and the inorganic scintillator crystals, without limitation on the volume of the detector.For future applications,increased dot density and optimized surface treatments should improve the light output by at least a fac-tor of20,and efficient photon collection using APDs should increase the number of photons counted by a factor of10. However,in order to accommodate the increased dot density, the Stokes shift will have to be increased to limit reabsorp-tion losses.Our next step is therefore to engineer the Stokes shift of semiconductor quantum dots.We will do this by anchoring chemical groups at the surface of the quantum dots,17or by using different semiconductor materials such as PbS.This work was performed under the auspices of the U.S. Department of Energy by University of California Lawrence Livermore National Laboratory under Contract No.W-7405-ENG-48.It was also supported by a Laboratory Directed Research and Development grant͑Grant No.04-ERD-107͒. 1C.B.Murray,D.J.Norris,and M.G.Bawendi,J.Am.Chem.Soc.115, 8706͑1993͒.2X.Peng,M.C.Schlamp,A.V.Kadavanich,and A.P.Alivisatos,J.Am. Chem.Soc.119,7019͑1997͒.3S.Kim,Y.T.Lim,E.G.Soltesz,A.M.De Grand,J.Lee,A.Nakayama, J.A.Parker,T.Mihaljevic,urence,D.M.Dor,L.H.Cohn,M.G. Bawendi,and J.V.Frangioni,Nat.Biotechnol.22,93͑2004͒.4I.L.Medintz,A.R.Clapp,H.Mattoussi,E.R.Goldman,B.Fisher,and J. M.Mauro,Nat.Mater.2,630͑2003͒.5Y.Chan,J.S.Steckel,P.T.Snee,J.-M.Caruge,J.M.Hodgkiss,D.G. Nocera,and M.G.Bawendi,Appl.Phys.Lett.86,73102͑2005͒.6D.E.Persyk,M.A.Schardt,T.E.Moi,K.A.Ritter,and G.Muehllehner, IEEE Trans.Nucl.Sci.27,168͑1980͒.7L. A.Andryushchenko, B.V.Grinev, A.M.Litichevskii,and L.V. Udovichenko,Instrum.Exp.Tech.40,59͑1997͒.8M.Moszynski,M.Balcerzyk,W.Czarnacki,M.Kapusta,W.Klamra,P. Schotanus,A.Syntfeld,and M.Szawlowski,IEEE Trans.Nucl.Sci.50, 767͑2003͒.9W.H.Berninge,IEEE Trans.Nucl.Sci.21,374͑1974͒.10M.Itaya,T.Inagaki,T.Iwata,G.I.Lim,H.Oishi,H.Okuno,Y.Tajima, H.Y.Yoshida,and Y.Yoshimura,Nucl.Instrum.Methods Phys.Res.A 522,477͑2004͒.11M.Akiba,M.Fujiwara,and M.Sasaki,Opt.Lett.30,123͑2005͒.12S.Dai,S.Saengkerdsub,H.-J.Im,A.C.Stephan,and S.M.Mahurin,AIP Conf.Proc.632,220͑2002͒.13J.F.Ziegler,J.P.Biersack,and U.Littmark,The Stopping and Range of Ions in Solids͑Pergamon,New York,1985͒.14G.F.Knoll,T.F.Knoll,and T.M.Henderson,IEEE Trans.Nucl.Sci.35, 872͑1988͒.15J.B.Birks,The Theory and Practice of Scintillation Counting͑Pergamon, New York,1967͒.16P.Dorenbos,J.T.M.de Haas,and C.W.E.van Eijk,IEEE Trans.Nucl. Sci.42,2190͑1995͒.17A.Puzder,A.J.Williamson,J.C.Grossman,and G.Galli,J.Am.Chem. Soc.125,2786͑2003͒.Reuse of AIP Publishing content is subject to the terms at: https:///authors/rights-and-permissions. Download to IP: 202.195.129.247 On: Tue, 19 Apr2016 13:32:35。
Biodegradable luminescent porous silicon nanoparticles for in vivo applications

PUBLISHED ONLINE: 22 FEBRUARY 2009 DOI: 10.1038/NMAT2398
Biodegradable luminescent porous silicon nanoparticles for in vivo applications
Ji-Ho Park1,2, Luo Gu1, Geoffrey von Maltzahn3, Erkki Ruoslahti4, Sangeeta N. Bhatia3,5,6
and Michael J. Sailor1,2,7*
Nanomaterials that can circulate in the body hold great potential to diagnose and treat disease1–4. For such applications, it is important that the nanomaterials be harmlessly eliminated from the body in a reasonable period of time after they carry out their diagnostic or therapeutic function. Despite efforts to improve their targeting efficiency, significant quantities of systemically administered nanomaterials are cleared by the mononuclear phagocytic system before finding their targets, increasing the likelihood of unintended acute or chronic toxicity. However, there has been little effort to engineer the self-destruction of errant nanoparticles into non-toxic, systemically eliminated products. Here, we present luminescent porous silicon nanoparticles (LPSiNPs) that can carry a drug payload and of which the intrinsic near-infrared photoluminescence enables monitoring of both accumulation and degradation in vivo. Furthermore, in contrast to most optically active nanomaterials (carbon nanotubes, gold nanoparticles and quantum dots), LPSiNPs self-destruct in a mouse model into renally cleared components in a relatively short period of time with no evidence of toxicity. As a preliminary in vivo application, we demonstrate tumour imaging using dextran-coated LPSiNPs (D-LPSiNPs). These results demonstrate a new type of multifunctional nanostructure with a low-toxicity degradation pathway for in vivo applications.
2000 novel processing of solar cells with porous silicon texuring
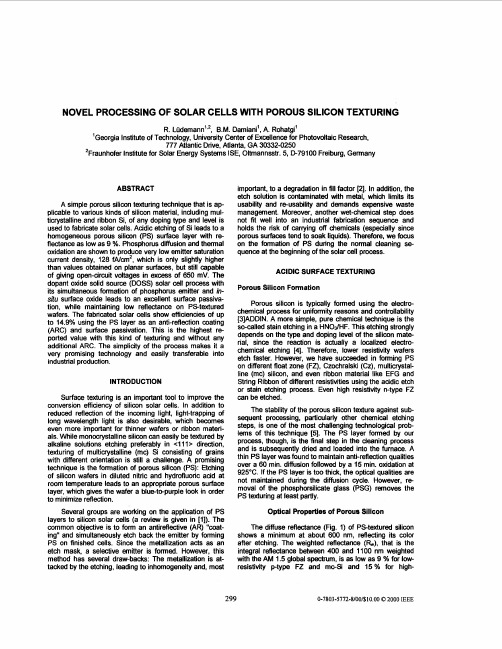
important, to a degradation in fill factor [ ] In addition, the 2. etch solution is contaminated with metal, which limits its usability and reusability and demands expensive waste management. Moreover, another wet-chemical step does not fit well into an industrial fabrication sequence and holds the risk of carrying off chemicals (especially since porous surfaces tend to soak liquids). Therefore, we focus on the formation of PS during the normal cleaning s e quence at the beginning of the solar cell process. ACIDIC SURFACE TEXTURING Porous Silicon Formation Porous silicon is typically formed using the electrochemical process for uniformity reasons and controllability [3]ADDIN. A more simple, pure chemical technique is the so-called stain etching in a HNOdHF. This etching strongly depends on the type and doping level of the silicon material, since the reaction is actually a localized electrochemical etching [4]. Therefore, lower resistivity wafers etch faster. However, we have succeeded in foning PS on different float zone (FZ), Czochralski (Cz), multicrystalline (mc) silicon, and even ribbon material like EFG and String Ribbon of different resistivities using the acidic etch or stain etching process. Even high resistivity n-type FZ can be etched. The stability of the porous silicon texture against subsequent processing, particularly other chemical etching steps, is one of the most challenging technological problems of this technique [5]. The PS layer formed by our process, though, is the final step in the deaning process and is subsequently dried and loaded into the furnace. A thin PS layer was found to maintain anti-reflection qualities over a 60 min. diffusion followed by a 15 min. oxidation at 925°C. If the PS layer is too thick, the optical qualities are not maintained during the diffusion cycle. However, r e moval of the phosphorsilicate glass (PSG) removes the PS texturing at least partly. Optical Properties of Porous Silicon The diffuse reflectance (Fig. 1) of PStextured silicon shows a minimum at about 600 nm, reflecting its color after etching. The weighted reflectance (Rw), that is the integral reflectance between 400 and 1100 nm weighted with the AM 1.5 global spectrum, is as low as 9 % for lowresistivity p-type FZ and mo-Si and 15 % for high-
嵌入式3D打印多孔硅胶义眼台及其表面修饰

眼部的重度创伤、严重感染、重度眼球萎缩、恶性肿瘤晚期等均可能造成眼球不可逆损伤,常需要将病变眼球摘除[1]。
眼球摘除后不仅导致患者病变侧视力缺失,还会影响面容美观,给患者造成巨大的心理负担[2]。
义眼台可代替眼窝体积、改善面部容貌,成为眼球缺失后整形修复的首选[3]。
羟基磷灰石和高密度聚乙烯是义眼台的常用材料,但二者造价昂贵且力学强度明显高于眼周组织,植入术后常引发出血、感染、眼周组织坏死等并发症[3,4]。
硅胶是一种生物相容性良好且价格低廉的弹性材料,更接近人体眼周组织的力学强度(0.72~1.28Mpa ),用于义眼台制备不仅降低了制造成本且可有效避免上述并发症[5-7]。
但硅胶的疏水特性不利于植入后的细胞组织黏附,无孔硅胶义眼台植入术后常伴随极高Embedded 3D printing of porous silicon orbital implants and its surface modificationZHAO Hong 1,2,WANG Yilin 2,WANG Yanfang 3,GONG Haihuan 2,YINJUN Feiyang 2,CUI Xiaojun 1,ZHANG Jiankai 1,HUANG Wenhua 1,21Department of Human Anatomy,School of Basic Medical Sciences,Guangdong Medical University,Dongguan Key Laboratory of Stem Cell and Regenerative Tissue Engineering,Dongguan 523808,China;2National Key Discipline of Human Anatomy,School of Basic Medical Sciences,Southern Medical University,Guangdong Provincial Key Laboratory of Digital Medical and Biomechanics,Guangdong Engineering Research Center for Translation of Medical 3D Printing Application,Guangzhou 510515,China;3Guangdong Provincial People's Hospital Affiliated to Southern Medical University,Guangdong Academy of Medical Science,Guangzhou 510080,China摘要:目的制备个性化多孔硅胶义眼台并探讨表面修饰对硅胶义眼台性能的影响。
微软硅胶雕刻系统快速参考卡说明书
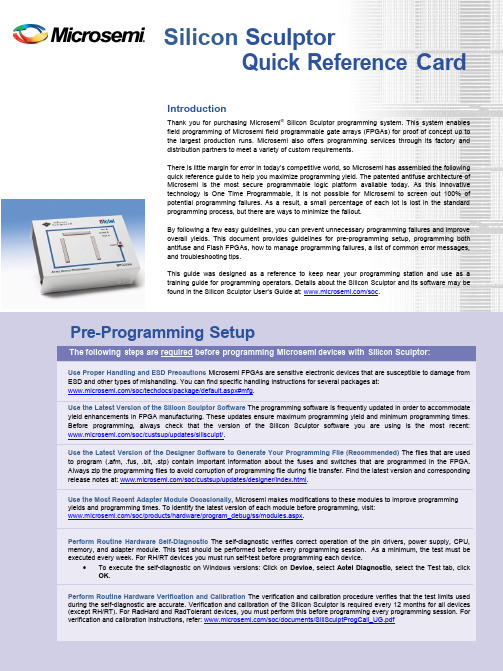
Silicon SculptorQuick Reference CardIntroductionThank you for purchasing Microsemi®Silicon Sculptor programming system. This system enables field programming of Microsemi field programmable gate arrays (FPGAs) for proof of concept up to the largest production runs. Microsemi also offers programming services through its factory and distribution partners to meet a variety of custom requirements.There is little margin for error in today’s competitive world, so Microsemi has assembled the following quick reference guide to help you maximize programming yield. The patented antifuse architecture of Microsemi is the most secure programmable logic platform available today. As this innovative technology is One Time Programmable, it is not possible for Microsemi to screen out 100% of potential programming failures. As a result, a small percentage of each lot is lost in the standard programming process, but there are ways to minimize the fallout.By following a few easy guidelines, you can prevent unnecessary programming failures and improve overall yields. This document provides guidelines for pre-programming setup, programming both antifuse and Flash FPGAs, how to manage programming failures, a list of common error messages, and troubleshooting tips.This guide was designed as a reference to keep near your programming station and use as a training guide for programming operators. Details about the Silicon Sculptor and its software may be found in the Silicon Sculptor User’s Guide at:/soc.Programming Programming Antifuse FPGAsBelow are the specific guidelines on what you can do when you encounter programming failures with Microsemi FPGAs.Antifuse FPGAs (non RH/RT)Any Time You Encounter a Failure:• Record the EXACT error message• Compare your error message to those listed in the “CommonProgramming Failure Modes” table, and try to resolve theproblem based on the given suggestions.If failures continue, proceed to the next step.2.•Record the version of the Silicon Sculptor software being used, then upgrade to the latest version:/soc/custsup/updates/silisculpt•Perform the self-diagnostic•Record the exact part number of the adapter mod ule(s) being used, then upgrade to the latest version:/soc/products/hardware/program_debug/ss/modules.aspxContinue programming and proceed to the next step.3.Compare your programming fallout with the “Programming FailureAllowance Table” located to the right. As long as you are within the guidelines, continue programming. Contact your distributor or sales office to return devices, and provide failure rates along with yourrequest.If the failure rate exceeds expected fallout, proceed to thenext step.4.Record the following for all failures andprogrammed devices:•Date code (4 digit number on top of device)•Lot code (alphanumeric usually on underside of device)•Number failed and number passed programmingfrom each lotRadHard and RadTolerant FPGAs1.Stop Programming Immediately.Due to the high cost of RH/RT devices, it is important that youensure your software and hardware are up to date and are in goodworking condition. It is also important that you provide detailedinformation about the failure to Microsemi. Refer to the “RH/RTProgramming Guide” and “RTAX-S/SL/DSP Programming Guide”application note on Microsemi website for more detailedinformation about programming RH and RT devices.•Record the EXACT error message•Save the .txt file under a different name, so it is not overwritten•Perform the self-diagnostic•Record the version of the Silicon Sculptor software beingused, then upgrade to the latest version:/soc/custsup/updates/silisculpt•Record the exact part number of the programmingmodule(s) being used, then upgrade to the latest version:If failures continue, proceed to the next step.2.Compare your programming fallout with the “Programming FailureAllowance Table” located to the right. As long as you are within theguidelines, continue programming. Contact your distributor or salesoffice to return devices, and provide ALL of the above informationwith your request.If the failure rate exceeds expected fallout, proceed to the nextstep.3.Record the following for all failures andprogrammed devices:•Date code (4 digit number on top of device)•Lot code (alphanumeric usually on underside of device)•Serial number (top of device)•Number failed and number passed programming from eachLotFlash FPGAsEven though Microsemi tests the programmability of e veryFlash FPGA, there are several external fac tors that cancause devices to fail, especially when performing In-SystemProgramming, such as noise and out-of date software andprogram ming hardware. Review the application notes onMicrosemi website for details on ISP:/soc/techdocs/appnotes/default.aspxFor all failures:•Record the EXACT error message•Record the operation being executed when error occurs•Compare your error message to those listed in this table,and try to resolve the problem based on the suggestionsgiven.If failures continue, proceed to the next step.2.•Record the version of the Silicon Sculptor software being used, thenupgrade to the latest version:/soc/custsup/updates/silisculpt•Perform the self-diagnostic•Record the exact part number of the pr ogramming module(s) beingused, then upgrade to the latest version:/soc/documents/FA_Policies_Guidelines_5-06-00002.pdfIf failures continue, proceed to the next step.Record the following for all failures and programmeddevices:•Date code (4 digit number on top of device)•Lot code (alphanumeric usually on underside of device)•Number failed and number passed programming from each lot“Programming andFunctional FailureGuidelines”Contact Microsemi Support with the Results of the Above Diagnostics Including:Antifuse∙Programming FA checklist(/soc/documents/Programming_Failure_Analysis_Checklist_Antifuse.zip) ∙Programming log files (C:\BP\DATALOG)∙Afm and adb files∙Specific Error Messages Obtained∙The .log File RH/RT∙Programming FA checklist(/soc/documents/Programming_Failure_Analysis_Checklist_Antifuse.zip)∙Programming log files: (C:\BP\DATALOG)∙Afm and adb files∙Software Versions Used∙Adapter Module Part NumberFlash∙Programming FA checklist (/soc/documents/Programming Failure_Analysis_Checklist_Flash.zip)∙Programming log files (C:\BP\DATALOG)∙Stp and adb filesCommon Programming Failure ModesNote (RH/RT only):Before programming any additional RH/RT devices, first complete steps 1 and 2 of the previous page. Error Probable Cause ResolutionInvalid Electronic Signature in Chip (Device ID) The chip may be damaged. Try to program another device.The chip is not in the proper position inthe programmer site.Reseat the device to ensure that it is oriented correctly in the socketand is making good contact with the socket.The socket is dirty and not making aconnection.Clean the socket and make sure that it is making good contact withthe device.Device is Not BlankThe device was previously programmedand cannot be erased. The Device/Blank command was executed or the “Blank check before programming” option was enabled in the Device/Options dialog box, and the device in the programmer site is determined to have programmed data.The wrong algorithm was used. Make sure that the device and package selected in the softwareexactly matches the device being programmed.Incorrect Checksum Previous programming is done on devicewith different programming file. Make sure the AFM file used to perform the Microsemi_Checksum command is the AFM file used to program the device.The checksum fuses were not programmed correctly. The programmer has reported that the checksum antifuses could not be programmed. Record the actual and reported checksum values, and continue following the guidelines for handling programming failures.Failed to Program Fuse XXX Pulse YYY There is a problem programming thisparticular antifuse.The programmer has reported that this antifuse cannot beprogrammed. Record the fuse and pulse numbers, and continuefollowing the guidelines for handling programming failures.AFM programming file corruption. It may be due to improper file transfer (always zip the programmingfile before transferring).Failed Current SenseThe standby current has increasedsignificantly after programming. The programmer has reported that the device cannot be reliably programmed. Record the error message, and continue following the guidelines for handling programming failures.Failed Fuse XXX,Integrity Test YYY The programmer has reported that thisdevice cannot be correctly programmed. XXX indicates the fuse number failed. YYY indicates the test number. Record the fuse and integrity test numbers, and continue following the guidelines for handling programming failures.Excessive Current Detected. The Protection Circuit Has Shut Off the Power The command was aborted to protect theprogrammer and the device. The devicewas taking too much current from theprogrammer.Reseat the device to ensure that it is oriented correctly in the socketand is making good contact with the socket.The wrong algorithm could be selectedand improper voltages were applied tothe chip in the programmer site.Select the correct device and package in the Silicon Sculptorsoftware, and make sure you are using the latest version of theprogramming software.There could be a short in theprogramming adapter.Perform the self-diagnostic with the module on the programmer, andtry another module if available.The device is damaged.Remove the chip and run the self-diagnostic to make sure all the pindrivers are functioning correctly. If the hardware passes the test, besure you have the correct algorithm (device entry) selected for yourdevice. If the error still occurs and you are sure the device is insertedcorrectly, then the problem may be a faulty device.Error in Programming Algorithm The software has detected an internalerror.Download the latest Silicon Sculptor software and try again.There is No Data in the Buffer. You Must Load a File A command tried to read data from thebuffer to program or verify a chip, butnothing has been loaded into the bufferyet or the buffer was recently cleared.Open the programming file you wish to use in the Silicon Sculptorsoftware.AFM programming file corruption. It may be due to improper file transfer (always zip the programmingfile before transferring)Microsemi Corporate HeadquartersOne Enterprise, Aliso Viejo CA 92656 USA Within the USA: +1 (949) 380-6100 Sales: +1 (949) 380-6136 Fax: +1 (949) 215-4996。
磁控溅射薄膜生长的模拟方法

表面技术第51卷第2期磁控溅射薄膜生长的模拟方法王晓倩,赵晋,刘建勇(天津工业大学 纺织科学与工程学院,天津 300387)摘要:磁控溅射技术制备的薄膜膜层均匀,内部无气孔,密度高,与衬底的附着性良好,薄膜质量高,被广泛应用于科学研究和工业生产中,且适合应用计算机模拟来研究溅射过程和溅射结果,这样既可以检验模拟的准确性,又可以对实验现象的内在意义进行挖掘,为后续实验提供参考信息。
在介绍磁控溅射薄膜生长常用模拟方法原理的基础上,详细讨论了第一性原理(First-principles calculations)、分子动力学(Molecular dynamics,MD)和蒙特卡洛(Monte Carlo,MC)等3种方法的适用条件和模拟结果,从3种方法适合解决的问题、相互之间的区别等方面,对国内外最新的研究进展进行总结与分析。
发现3种方法在精确度和计算量上依次递减,在可模拟的时间和空间尺度上依次递增,在模拟对象上,第一性原理方法由于其高度的精确性被广泛应用于对薄膜本身的性质或对粒子间的运动等方面,且模拟结果可以是具体数值,从而对实验进行更加精确的预测和指导,分子动力学方法多用于模拟薄膜生长过程和原子间行为等方面,蒙特卡洛方法相较于前两者,用途更加广泛,可模拟的对象除了薄膜本身,也可以对电磁场等进行模拟。
最后,对磁控溅射薄膜生长模拟未来的研究方向进行了展望。
关键词:磁控溅射;第一性原理方法;分子动力学方法;蒙特卡洛方法;数值模拟;薄膜中图分类号:O484 文献标识码:A 文章编号:1001-3660(2022)02-0156-09DOI:10.16490/ki.issn.1001-3660.2022.02.014Simulation Method of Magnetron Sputtering Film GrowthWANG Xiao-qian, ZHAO Jin, LIU Jian-yong(School of Textile Science and Engineering, Tiangong University, Tianjin 300387, China)ABSTRACT: The film prepared by magnetron sputtering technology is uniform and free from pores inside, with high density, good adhesion to the substrate and excellent quality and has been widely used in scientific research and industrial production.Such film is suitable for the simulation by computer to study sputtering process and sputtering results, which can not only check the accuracy of simulation, but also excavate the intrinsic meaning of experimental phenomena and provide reference information for subsequent experiments. Based on the introduction of the principles of the commonly used simulation methods for the growth of magnetron sputtering films, the applicable conditions and simulation results of the three methods, first-principles calculations, molecular dynamics (MD) and Monte Carlo (MC), were discussed in detail and the latest research收稿日期:2020-09-02;修订日期:2021-07-12Received:2020-09-02;Revised:2021-07-12作者简介:王晓倩(1995—),女, 硕士研究生,主要研究方向为纺织品功能整理。
现代CMOS工艺基本流程(英文)

N Well
-
P Well Silicon Epi Layer P Silicon Substrate P+ 18
-
3. Gate Formation
Grow Sacrificial Oxide: A thin (~250Å) oxide layer is grown to capture defects in the silicon surface.
Future NMOS Transistor
Silicon Epi Layer P
-
Silicon Substrate P+ 14
Well Formation
Pattern Photoresist for P-Well Formation: A noncritical masking layer, utilizing thicker resist to block the implant.
Basic CMOS Process Flow 现代CMOS工艺基本流程
1
Basic CMOS Process Flow
~2 microns
Silicon Epi Layer P
-
Starting Point: Pure silicon wafer (heavily-doped) with a lightlydoped epitaxial (epi) layer. An epi layer is used to provide a cleaner layer for device formation and to prevent “latch-up” of CMOS transistors.
Pad Oxide
Silicon Epi Layer P
LCD专业翻译
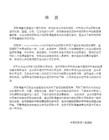
The Field Effect type TFT with amorphous silicon material contains three terminal components of three types of electrodes: source, drain, and gate. They are often used as the switch of active matrix type LCD.
Aperture ratio
ቤተ መጻሕፍቲ ባይዱ
单一画素中可透光面积与画素总面积之比值。开口率之大小与TFT所使用之金属导线特性及Cell制程能力有关。
英:
It is the ratio of the luminary surface in a single pixel comparing to the surface of total pixels. The opening rate is determined by the nature of metal interconnect and the capability of Cell manufacturing of TFT.
在画素或dot上设置主动组件,于写入期间激活主动组件而写入data电压、其它期间则关闭主动组件以维持电压之矩阵驱动方式。依据主动组件的种类区分为3端子型的TFT方式与2端子型的TFD(MIM)方式。2端子型的制造工程可简化。
英:
It sets active components on pixel or dot, witch activate active component to write data voltage during the writing period, and during other period, it shut off active component to maintain voltage of matrix driving method. According to different types of active component, it is divided into either 3-terminal model TFT or 2-terminal model TFD (MIM) methods. The manufacturing process of 2-terminal model can be simplified.
Doping silicon wafers by use of silicon paste
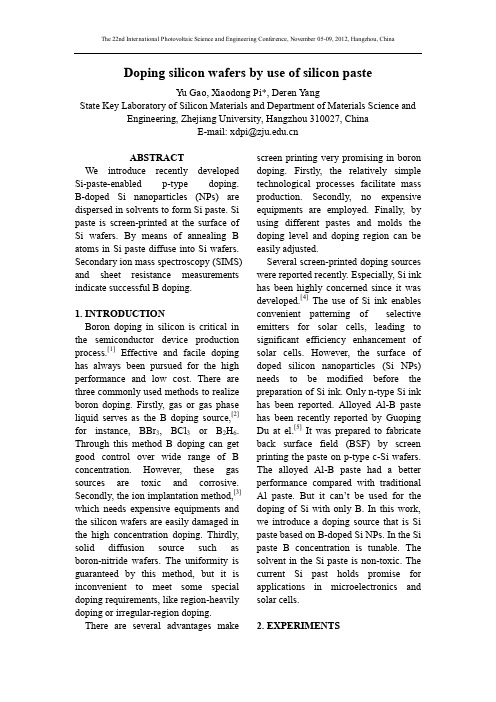
Doping silicon wafers by use of silicon pasteYu Gao, Xiaodong Pi*, Deren YangState Key Laboratory of Silicon Materials and Department of Materials Science and Engineering, Zhejiang University, Hangzhou 310027, ChinaE-mail: xdpi@ABSTRACTWe introduce recently developed Si-paste-enabled p-type doping. B-doped Si nanoparticles (NPs) are dispersed in solvents to form Si paste. Si paste is screen-printed at the surface of Si wafers. By means of annealing B atoms in Si paste diffuse into Si wafers. Secondary ion mass spectroscopy (SIMS) and sheet resistance measurements indicate successful B doping.1. INTRODUCTIONBoron doping in silicon is critical in the semiconductor device production process.[1]Effective and facile doping has always been pursued for the high performance and low cost. There are three commonly used methods to realize boron doping. Firstly, gas or gas phase liquid serves as the B doping source,[2] for instance, BBr3, BCl3or B2H6. Through this method B doping can get good control over wide range of B concentration. However, these gas sources are toxic and corrosive. Secondly, the ion implantation method,[3] which needs expensive equipments and the silicon wafers are easily damaged in the high concentration doping. Thirdly, solid diffusion source such as boron-nitride wafers. The uniformity is guaranteed by this method, but it is inconvenient to meet some special doping requirements, like region-heavily doping or irregular-region doping. There are several advantages make screen printing very promising in boron doping. Firstly, the relatively simple technological processes facilitate mass production. Secondly, no expensive equipments are employed. Finally, by using different pastes and molds the doping level and doping region can be easily adjusted.Several screen-printed doping sources were reported recently. Especially, Si ink has been highly concerned since it was developed.[4]The use of Si ink enables convenient patterning of selective emitters for solar cells, leading to significant efficiency enhancement of solar cells. However, the surface of doped silicon nanoparticles (Si NPs) needs to be modified before the preparation of Si ink. Only n-type Si ink has been reported. Alloyed Al-B paste has been recently reported by Guoping Du at el.[5]It was prepared to fabricate back surface field (BSF) by screen printing the paste on p-type c-Si wafers. The alloyed Al-B paste had a better performance compared with traditional Al paste. But it can’t be used for the doping of Si with only B. In this work, we introduce a doping source that is Si paste based on B-doped Si NPs. In the Si paste B concentration is tunable. The solvent in the Si paste is non-toxic. The current Si past holds promise for applications in microelectronics and solar cells.2. EXPERIMENTSBoron-doped Si NPs were synthesized by a plasma approach.[6]The B concentration of Si NPs was analyzed by chemical titration. An X-ray diffraction equipment (Panalytical X'Pert PRO) was employed to study the microstructure of Si NPs. Czochralski (CZ) Si wafers used in this work were phosphorus-doped with a resistivity of 1.5–3 Ω/cm.Si NPs were dispersed in terpineol with a weight percentage of 30%. The resulting paste was screen-printed on Si wafers and then heated in a tube furnace at 900℃for 1 h in the atmosphere of nitrogen. After cleaning Si wafers were examined with secondary ion mass spectroscopy (SIMS) and four-point probe.3. RESULTS AND DISCUSSIONS Figure 1 shows the XRD spectra of Si NPs with different B concentrations. We find that all the XRD peaks are related to Si crystal. The three strongest peaks at ~28.5, 47.4 and 56.3 degrees correspond to (111), (220) and (311), respectively. Generally speaking, the intensity values of XRD peaks are not comparable because the peak intensity is related to the amount of tested sample. In this work the amount of each sample was enough to lead to the saturation of XRD signals. All the samples were measured under the same conditions. Therefore, qualitative analysis of peak intensity is reasonable. It is found that the increase of B concentration leads to the decrease of the peak intensity and the increase of the full width at half maximum (FWHM). When B concentrations are 18%, 31%, 39% and 42%, the FWHMs of (111) peaks are 0.414°, 0.466°, 0.492°and 0.588°, respectively. The changes of peak intensity and FWHM indicate that the crystallinity deteriorates as B concentration increases. This may be because the incorporated B atoms seriously affect the Si lattice. By analyzing the broadening of the XRD peaks we found that all the Si NPs areabout 20 nm large.Fig. 1 XRD spectra from Si NPs with B concentrations of 18%, 31%, 39% and 42%.Figure 2 shows the distribution of B along the depth. When B concentrations in Si NPs are 18%, 31%, 39% and 42%, the highest B concentrations near the wafer surface are about 1.6 × 1020, 2.7 ×1020, 6.7 ×1020and 1.0 ×1021 atoms/cm3, respectively. The amount of B diffused inside Si wafers is proportional to the B concentration in the paste. We can control the doping level over a wide range by choosing Si NPs with different B concentrations.Fig. 2B doping profiles in n-type Si wafers (after heating at 900℃for 1h). The concentration of B in Si NPs varies from 18 to 42%.Table 1 shows the sheet resistances of Si wafers that are doped with Si paste. The sheet resistance decreases with the increase of B concentration in Si NPs. When B concentrations in Si NPs are 18%, 31%, 39% and 42%, the sheet resistances are 193, 173, 114 and 84Ω/□, respectively. This variation is consistent with the SIMS results. Sheet resistance is determined by the B concentration and B diffusion depth in a Si wafer. B diffusion depth is essentially the same in this work, as indicated in Figure 2. The use of Si paste with high B concentration leads to high B concentration in a wafer, resulting in a low sheet resistance of the wafer.Table 1.Sheet resistances of Si wafers doped with Si paste. The concentration4. CONCLUSIONSSi paste has been prepared by using B-doped Si NPs. The doping of Si wafers is performed by screen-printing Si paste at the wafer surface. The doping level can be adjusted by using Si paste with different B concentrations. B concentrations near the wafer surface are in the range from 1.6×1020to 1.0×1021 when the concentration of B in Si NPs used for Si paste changes from 18% to 42%. The Si paste enabled doping leads to varying sheet resistances that are suitable for device fabrication. REFERENCES[1] A. Wang, J. Zhao and M. A. Green, Appl. Phys. Lett.57, 602 (1990).[2] A. Kessler, T. Ohrdes, M. B. Wolpensinger and Nils-Peter Harder, Semicond. Sci. Technol.25, 055001 (2010).[3] Y. Lu and A. Lal, Nano lett. 10, 4651 (2010).[4] H. Antoniadis, Proceedings of the 34th IEEE Photovoltaic Specialist Conference, Philadelphia, USA(2010).[5] G. P. Du, B. Chen, N. Chen and R. Y. Hu, IEEE Electr Device Lett.33, 573 (2012).[6] X. D. Pi, Q. Li, D. S. Li and D.R. Yang, Sol. Energ. Mater. Sol. Cells95, 2941 (2011)。
半导体行业专业英语名词解释

缺陷分析软件
157
SEM
(SCANNING ELECTRON MICROSCOPE)
电子显微镜
158
SELECTIVITY
选择性
159
SILICIDE
硅化物
160
SILICIDE
金属硅化物
161
SILICON
硅
162
SILICON NITRIDE
氯化硅
二氟化硼
20
BOAT
晶舟
21
B.O.E
缓冲蚀刻液
22
BONDING PAD
焊垫
23
BORON
硼
24
BPSG
含硼及磷的硅化物
25
BREAKDOWN VOLTAGE
崩溃电压
26
BURN IN
预烧试验
27
CAD
计算机辅助设计
28
CD MEASUREMENT
微距测试
29
CH3COOH
醋酸
30
CHAMBER
真空室,反应室
(TI MEMORY PROTOTYPE,TMS-X TI MEMORY STANDARD PRODUCT)
TI记忆产品样品(原型),TI内存标准产品
184
TOX
氧化层厚度
185
TROUBLE SHOOTING
故障排除
186
UNDERCUT
底切度
187
UNIFORMITY
均匀度
188
VACUUM
真空
系统暂时性失效比率测试
173
STEP COVERAGE
SILICON SM5812 5852 Datasheet

SILICONMICROSTRUCTURES I N C O R P O R A T E DSM5812/SM5852Amplified Pressure Sensor• N OW WITH ACCESS TO D IGITAL T EMPERATURE AND C ORRECTED D IGITAL P RESSURE • L OW -COST , FULLY AMPLIFIED , CALIBRATED , AND COMPENSATED IN A SINGLE PACKAGE• A VAILABLE FOR D IFFERENTIAL , S INGLE -ENDED DIFFERENTIAL , G AGE & A BSOLUTE APPLICATIONS •M ULTIPLE PRESSURE RANGES AVAILABLE TO MEASURE PRESSURE DOWN TO 0.15 PSI FULL -SCALEAND UP TO 100 PSI FULL -SCALEDESCRIPTIONThe Silicon Microstructures SM5812 and SM5852 series of OEM pressure sensors combines state-of-the-art pressure sensor technology with CMOS digital signal processing technology to produce an amplified, fully conditioned, multi-order pressure and temperature compensated sensor in a dual in-line package (DIP) configuration.Combining the pressure sensor with acustom signal conditioning ASIC in a single package simplifies the use of advanced silicon micromachined pressure sensors. Now, the pressure sensor can be mounted directly to a standard printed circuit board and an amplified, high level, calibrated pressure signal can be acquired from the digital interface or analog output. This eliminates the need of additional circuitry, such as a compensation network or micro-controller containing a custom correction algorithm.The SM5812/SM5852 Series pressure sensors are based on SMI's highly stable, piezoresistive pressure sensor chips mounted on a ceramic substrate. An electronically programmable ASIC iscontained in the same package to provide calibration and temperature compensation.The model SM5812 is designed foroperating pressure ranges from 0-5 PSI up to 0-100 PSI. The model SM5852 isdesigned for operating pressure ranges from 0-0.15 PSI up to 0-3 PSI. For both models, the sensor output is ratiometric with the supply voltage.FEATURES• Amplified, calibrated, fully signalconditioned output span of 4.0 VDC FS (0.5 to 4.5 V signal)• Digital temperature and calibratedpressure available through I 2C interface • Output ratiometric with supply voltage • Multi-order correction for pressure non-linearity (factory programmed)• Multi-order correction for temperature coefficient of span and offset (factory programmed)• Gage, differential, and absolute versions •SMI’s unique low-pressure die allows for a full-scale pressure range of 0-0.15 PSITYPICAL APPLICATIONS• Barometric measurement • Medical instrumentation • Pneumatic control • Gas flow• Respirators and ventilators•Heating, Ventilation and Air Conditioning (HVAC)查询SM5812-005-A-3-L供应商SM5812/SM5852THEORY OF OPERATIONThe operation of the signal processor is depicted in the block diagram below. The external pressure sensor is a piezoresistive bridge. This transduces the applied pressure into an electronic signal, which is then inputted into the integrating amplifier of the ASIC. During the amplification step an offset correction factor is added in order to allow maximum gain for a given pressure while minimizing the offset error.The signal is then passed to an 11-bit analog to digital converter (ADC). The ADC samples the signal multiple times and uses the sum of those samples as a 13-bit word.A digital signal processor (DSP) is then used to correct and calibrate the pressure signal. The DSP provides multi-order correction of both pressure and temperature non-linearity through the use of factory-programmed coefficients. A combined total of twenty coefficients are available for correcting pressure and temperature non-linearity. The unique coefficients are determined during a calibration process performed at the factory. Factory calibration is the last step performed which means the effect of the package on the pressure signal will also be taken into account. This provides a great advantage over conventional laser-trimming approaches.The DSP outputs a corrected digital word, which travels to a 12-bit digital to analog converter (DAC) to provide a calibrated analog output. In addition to the analog output, the corrected pressure signal is accessible through an I2C digital interface. See SMI application note AN05-001 for a detailed description of how to read out the digital corrected pressure signal using theI2C bus interface.Analog OutputSDA SCLSILICONMICROSTRUCTURES I N C O R P O R A T E DSM5812/SM5852CHARACTERISTICS FOR SM5812/SM5852 – SPECIFICATIONSAll parameters are measured at room temperature while applying 5.000V supply, unless otherwise specified.Absolute 1, Gage & Single 2DifferentialMIN TYP MAX MIN TYP MAX UNITS NOTES Zero output (absolute and gauge) 0.42 0.50 0.58 V 3 Zero output (differential)2.422.502.58V3Output Span3.924.00 4.08 1.96 2.00 2.04 V FS 3, 40.15 PSI 3.80 4.00 4.20 1.90 2.00 2.10 V FS 3, 4 Linearity -0.5 +0.5 -0.5 +0.5 %FS 5 0.15 PSI-2.5+2.5-2.5+2.5%FS5 Pressure hysteresis 5812 -0.1 +0.1 -0.1 +0.1 %FS5852 -0.3+0.3 -0.3 +0.3 %FS Temperature coefficient - Zero-1.0 +1.0 -1.0 +1.0 %FS 0.15 PSI-2.5 +2.5 -2.5 +2.5 %FS Temperature coefficient – Span-1.0 +1.0 -1.0 +1.0 %FS 0.15 PSI-2.0 +2.0 -2.0 +2.0 %FS Thermal hysteresis -0.1 +0.1 -0.1 +0.1 %FS Response Time 2 2 msec Supply voltage 4.75 5.00 5.25 4.75 5.00 5.25 V 3, 7Current consumption10 10 mAOverpressure 5812 3X 3X %FS 65852 15X15X %FS 6 Operating temperature range -40 25 +125 -40 25 +125 °C Compensated temperature range 0 25 +70 0 25 +70 °CStorage temperature range -5525+135-5525+135°CMedia compatibility 8Weight 3 3 gramNotes: 1. Absolute parts are only offered in the SM5812 Series.2. Single-ended parts (Pressure Type - S) have 2 ports and are for higher gain differential applications where the differential pressure is always positive.3. Sensor output is ratiometric to supply.4.Full-scale (FS) is defined as zero pressure to rated pressure; differential parts can be used ±FS. Absolute and Gauge zero output is 0.5 V typical and full-scale output is 4.5 V. Span is the difference between Full-scale output and zero output, (4 V). For Differential parts, the negative full-scale is typically at 0.5 V, zero is typically 2.5 V, and positive full-scale is 4.5 volts to give a span of ±2.0 V.5. Defined as best fit straight line for positive pressure applied to the part6. Or 225 PSI, whichever is less. Output amplifier will saturate at about 0.25 V for applied pressure below the rated Zero and at about 4.75 V for applied pressure above the rated Full-scale.7. A 100 nF filter capacitor must be placed between Vsupply and Ground.8.Clean, dry gas compatible with wetted materials. Wetted materials include Pyrex glass, silicon, alumina ceramic, epoxy, RTV, gold, aluminum, and nickel.SM5812/SM5852ADDITIONAL INFORMATIONFull-Scale Pressure RangesSM5812 PSI [kPa] SM5852 PSI [kPa]005 5 [34.5] 001 0.15 [1.0] 015 15 [103.4]003 0.30 [2.1] 030 30 [206.8]008 0.80 [5.5] 060 60 [413.7]015 1.50 [10.3] 100 100[689.5]030 3.00 [20.7]。
Effect of NH(4)OH Concentration on Surface Qualities of a Silicon Wafer after Final-Touch Polishing
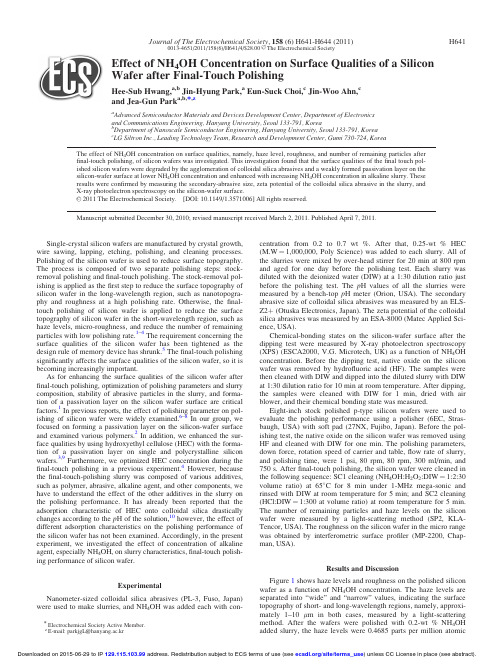
Effect of NH4OH Concentration on Surface Qualities of a Silicon Wafer after Final-Touch PolishingHee-Sub Hwang,a,b Jin-Hyung Park,a Eun-Suck Choi,c Jin-Woo Ahn,cand Jea-Gun Park a,b,*,za Advanced Semiconductor Materials and Devices Development Center,Department of Electronicsand Communications Engineering,Hanyang University,Seoul133-791,Koreab Department of Nanoscale Semiconductor Engineering,Hanyang University,Seoul133-791,Koreac LG Siltron Inc.,Leading Technology Team,Research and Development Center,Gumi730-724,KoreaThe effect of NH4OH concentration on surface qualities,namely,haze level,roughness,and number of remaining particles afterfinal-touch polishing,of silicon wafers was investigated.This investigation found that the surface qualities of thefinal touch pol-ished silicon wafers were degraded by the agglomeration of colloidal silica abrasives and a weakly formed passivation layer on the silicon-wafer surface at lower NH4OH concentration and enhanced with increasing NH4OH concentration in alkaline slurry.These results were confirmed by measuring the secondary-abrasive size,zeta potential of the colloidal silica abrasive in the slurry,andX-ray photoelectron spectroscopy on the silicon-wafer surface.V C2011The Electrochemical Society.[DOI:10.1149/1.3571006]All rights reserved.Manuscript submitted December30,2010;revised manuscript received March2,2011.Published April7,2011.Single-crystal silicon wafers are manufactured by crystal growth, wire sawing,lapping,etching,polishing,and cleaning processes. Polishing of the silicon wafer is used to reduce surface topography. The process is composed of two separate polishing steps:stock-removal polishing andfinal-touch polishing.The stock-removal pol-ishing is applied as thefirst step to reduce the surface topography of silicon wafer in the long-wavelength region,such as nanotopogra-phy and roughness at a high polishing rate.Otherwise,thefinal-touch polishing of silicon wafer is applied to reduce the surface topography of silicon wafer in the short-wavelength region,such as haze levels,micro-roughness,and reduce the number of remaining particles with low polishing rate.1–4The requirement concerning the surface qualities of the silicon wafer has been tightened as the design rule of memory device has shrunk.5Thefinal-touch polishing significantly affects the surface qualities of the silicon wafer,so it is becoming increasingly important.As for enhancing the surface qualities of the silicon wafer after final-touch polishing,optimization of polishing parameters and slurry composition,stability of abrasive particles in the slurry,and forma-tion of a passivation layer on the silicon wafer surface are critical factors.1In previous reports,the effect of polishing parameter on pol-ishing of silicon wafer were widely examined.6–8In our group,we focused on forming a passivation layer on the silicon-wafer surface and examined various polymers.2In addition,we enhanced the sur-face qualities by using hydroxyethyl cellulose(HEC)with the forma-tion of a passivation layer on single and polycrystalline silicon wafers.3,9Furthermore,we optimized HEC concentration during the final-touch polishing in a previous experiment.4However,because thefinal-touch-polishing slurry was composed of various additives, such as polymer,abrasive,alkaline agent,and other components,we have to understand the effect of the other additives in the slurry on the polishing performance.It has already been reported that the adsorption characteristic of HEC onto colloidal silica drastically changes according to the p H of the solution,10however,the effect of different adsorption characteristics on the polishing performance of the silicon wafer has not been examined.Accordingly,in the present experiment,we investigated the effect of concentration of alkaline agent,especially NH4OH,on slurry characteristics,final-touch polish-ing performance of silicon wafer.ExperimentalNanometer-sized colloidal silica abrasives(PL-3,Fuso,Japan) were used to make slurries,and NH4OH was added each with con-centration from0.2to0.7wt%.After that,0.25-wt%HEC (M.W¼1,000,000,Poly Science)was added to each slurry.All of the slurries were mixed by over-head stirrer for20min at800rpm and aged for one day before the polishing test.Each slurry was diluted with the deionized water(DIW)at a1:30dilution ratio just before the polishing test.The p H values of all the slurries were measured by a bench-top p H meter(Orion,USA).The secondary abrasive size of colloidal silica abrasives was measured by an ELS-Z2þ(Otuska Electronics,Japan).The zeta potential of the colloidal silica abrasives was measured by an ESA-8000(Matec Applied Sci-ence,USA).Chemical-bonding states on the silicon-wafer surface after the dipping test were measured by X-ray photoelectron spectroscopy (XPS)(ESCA2000,V.G.Microtech,UK)as a function of NH4OH concentration.Before the dipping test,native oxide on the silicon wafer was removed by hydrofluoric acid(HF).The samples were then cleaned with DIW and dipped into the diluted slurry with DIW at1:30dilution ratio for10min at room temperature.After dipping, the samples were cleaned with DIW for1min,dried with air blower,and their chemical bonding state was measured.Eight-inch stock polished p-type silicon wafers were used to evaluate the polishing performance using a polisher(6EC,Stras-baugh,USA)with soft pad(27NX,Fujibo,Japan).Before the pol-ishing test,the native oxide on the silicon wafer was removed using HF and cleaned with DIW for one min.The polishing parameters, down force,rotation speed of carrier and table,flow rate of slurry, and polishing time,were1psi,80rpm,80rpm,300ml/min,and 750s.Afterfinal-touch polishing,the silicon wafer were cleaned in the following sequence:SC1cleaning(NH4OH:H2O2:DIW¼1:2:30 volume ratio)at65 C for8min under1-MHz mega-sonic and rinsed with DIW at room temperature for5min;and SC2cleaning (HCl:DIW¼1:300at volume ratio)at room temperature for5min. The number of remaining particles and haze levels on the silicon wafer were measured by a light-scattering method(SP2,KLA-Tencor,USA).The roughness on the silicon wafer in the micro range was obtained by interferometric surface profiler(MP-2200,Chap-man,USA).Results and DiscussionFigure1shows haze levels and roughness on the polished silicon wafer as a function of NH4OH concentration.The haze levels are separated into“wide”and“narrow”values,indicating the surface topography of short-and long-wavelength regions,namely,approxi-mately1–10l m in both cases,measured by a light-scattering method.After the wafers were polished with0.2-wt%NH4OH added slurry,the haze levels were0.4685parts per million atomic*Electrochemical Society Active Member. z E-mail:parkjgL@hanyang.ac.kr Journal of The Electrochemical Society,158(6)H641-H644(2011)0013-4651/2011/158(6)/H641/4/$28.00V C The Electrochemical SocietyH641(ppma)at for the wide values and 0.136ppma at for the narrow val-ues with large fluctuation from wafer to wafer,as shown in Fig.1a .With the increase of NH 4OH concentration,the roughness drasti-cally decreased until the addition of 0.6-wt %NH 4OH and saturated to about 0.11ppma at for the wide values and 0.025ppma at for the narrow values with a further increase of NH 4OH concentration.The roughness represents the surface topography in the micro-wave-length region and is divided into three different regions:1–25,25–80,and 80–250l m.The roughness values in these three wavelength regions show a similar trend to those of the haze levels.With the increase in NH 4OH concentration,the roughness in all of the meas-ured wavelength regions were drastically decreased and saturated to0.27,0.85,and 2.5A˚in the 1–25-,25–80-,and 80–250-l m regions,respectively,with over the addition of 0.5-wt %NH 4OH,as shown in Fig.1b .The number of remaining particles on the final touch-polished silicon wafer is shown in Fig.2.Particles larger than 45nm on the polished silicon wafers were measured.The number of remaining particles at 0.2-wt %NH 4OH is 34,361ea per 8-inch wafer and fluc-tuated largely from wafer to wafer.With increasing NH 4OH concen-tration,however,it was drastically decreased and saturated at about 160ea per 8-inch wafer with over the addition of 0.6-wt %NH 4OH and had a little fluctuation from wafer to wafer.The left insert in Fig.2shows an SP2map image of the polished silicon wafer at0.2-wt %NH 4OH,while the right side insert is that for 0.7-wt %NH 4OH.The SP2maps show that the number of remaining particles drastically reduces with increasing NH 4OH concentration.To reveal the reason for the enhancement of polishing performance with increasing NH 4OH concentration,we measured change in slurry characteristics,such as namely,p H,secondary abrasive size,and zeta potential of the colloidal silica abrasives in the slurry,and chemical composition at the silicon-wafer surface as a function of NH 4OH concentration.First,secondary abrasive size with and without the addition of HEC into the slurry was measured as a function of NH 4OH concen-tration,as shown in Fig.3.Without the addition of HEC into the slurry,the secondary abrasive size of colloidal silica is approxi-mately 55nm for all NH 4OH concentrations.However,with the addition of HEC into the slurry,the secondary abrasive size of col-loidal silica changed drastically as a function of NH 4OH concentra-tion.That is to say,it increased from 55nm in the case without HEC to 899nm in the case with HEC at 0.2-wt %NH 4OH.More-over,the secondary abrasive size in the case with HEC drastically decreased with increasing NH 4OH concentration and became satu-rated at 120nm with addition of 0.5-wt %NH 4OH.These results indicate that the increase of secondary abrasive size of colloidal silica abrasives is associated with the presence of HEC in the slurry.However,the drastic change of secondary abrasive size in accord-ance with NH 4OH concentration cannot be explained by measuring abrasive size only.Other slurry characteristics,namely,p H andzetaFigure 1.Surface topography on the final touch polished silicon wafer as a function of NH 4OH concentration:(a)haze levels and (b)roughness.Figure 2.(Color online)Number of remaining particles on the final-touch-polished silicon wafer as a function of NH 4OHconcentration.Figure 3.Secondary abrasive size with and without the addition of HEC into the slurry as a function of NH 4OH concentration.Journal of The Electrochemical Society ,158(6)H641-H644(2011)H642potential,of colloidal silica abrasives in the slurry were thus measured.Figure4a shows the zeta potential of colloidal silica abrasives in the slurry as a function of p H.As well known,the zeta potential of colloidal silica abrasives in the slurry has an isoelectric point(IEP) in the acid region,11,12and this fact coincides with the above-described result from our experiment.The zeta potential of colloidal silica abrasives gradually decreases with increasing p H according to Eq.1(Ref.11)SiÀOHþOHÀþHþ$SiÀOÀþH2OþHþ[1] In this experiment,the p H of the slurry increased with NH4OH concentration from10.37at0.2-wt%to10.81at0.7-wt%NH4OH, and the zeta potential gradually and negatively increased in that range,as shown in Fig.4b.As a result,as NH4OH concentration increased,the hydroxyl group(Si-OH)on the colloidal silica abra-sive in the slurry surface was further and further dissociated to Si-OÀaccording to Eq.1.However,the HEC in the slurry was adsorbed onto the colloidal silica abrasives in the slurry by hydro-gen bonding between the-O-(ether group)on the HEC and Si-OH on the colloidal silica surface.10It can thus be expected that the adsorption of HEC onto the colloidal silica abrasives decreases as NH4OH concentration increases;thus,the agglomeration of the col-loidal silica abrasives would be weakened as NH4OH concentration increases,as shown in Fig.3.Note that the agglomeration of colloi-dal silica abrasives is due to the largely adsorbed HEC on abrasives. This agglomerated colloidal silica abrasives in the slurry could de-grade surface roughness through the breakdown of agglomerated ab-rasive during the polishing process,13as shown in Fig.1.Although the agglomeration of colloidal silica abrasives enhanced by decreas-ing NH4OH concentration is one of the reasons for degradation of surface quality,it is not sufficient to explain the polishing results because HEC could be absorbed onto the silicon wafer.This absorp-tion is another key factor in reducing the number of remaining particles and surface roughness duringfinal-touch polishing.4To Figure4.Zeta potential of colloidal silica abrasive as a function of p H:(a)all p H range,(b)p H range from10to11.Figure5.(Color online)Chemical-bonding state on the silicon wafer meas-ured by XPS before and after dipping wafer into the slurry as a function ofNH4OH concentration:(a)Si2p spectra,(b)magnification of Si2p spectra,and(c)O1s spectra.Journal of The Electrochemical Society,158(6)H641-H644(2011)H643observe the adsorption characteristic of HEC onto the silicon-wafer surface,the chemical-bonding states of a silicon wafer(which depend on NH4OH concentration)were therefore measured by XPS.The silicon and oxygen spectra measured by XPS are shown in Fig.5.An HF-treated silicon wafer was measured as a reference sample.Figure5a shows the XPS spectra of silicon2p as a function of NH4OH concentration.A peak at99.3eV,which indicates Si-Si bonding,appeared in the reference sample.After the silicon wafer was dipped into the slurry,however,a peak at105.9eV appeared. This peak is not due to the formation of silicon oxide on the silicon wafer because4.4eV is the maximum shift due to the formation of silicon oxide on the silicon wafer.14After the silicon wafer was dipped into the HF solution,it was covered with Si-H and Si-F bonds,and they rapidly changed to Si-OH in the alkaline solution.15 In a previous report,we anticipated that the Si-OH bonding would be an adsorption site for HEC and confirmed that the adsorption of HEC onto the silicon wafer occurred.4Considering this evidence, we conclude that the peak at105.9eV indicates the adsorption of HEC onto the silicon wafer.To observe the difference of intensity according to NH4OH concentration at105.9eV,the binding-energy region from102to110eV in Fig.5a is magnified in Fig.5b.The peak intensity at105.9eV gradually increased with increasing NH4OH concentration and saturated at over0.6-wt%NH4OH.The peak intensity can be considered as an adsorbed amount of HEC onto the silicon wafer.It can therefore be interpreted that a thin HEC layer was formed on the silicon-wafer surface,and the amount of HEC absorbed on the surface increases as NH4OH concentration increases.The XPS spectra of oxygen1s are shown in Fig.5c. Although a peak at532.4eV for Si-O was detected in the reference sample without dipping the silicon wafer into the slurry,its intensity is weak owing to the strip of native oxide on the silicon wafer.With dipping the silicon wafer into the slurry,however,a peak appeared at534.9eV.The peak at534.9eV is also due to the adsorption of HEC onto the silicon-wafer surface.4The intensity of the oxygen peak is also lowest at0.2-wt%NH4OH and increased with increas-ing NH4OH concentration.It could be inferred that more adsorption of HEC on the silicon-wafer surface is originated from a larger amount of suspended HEC in the slurry within afixed amount of HEC due to less adsorption of HEC on the colloidal silica surface at higher NH4OH concentration. The result that the adsorption amount of HEC on the silicon wafer increased as NH4OH concentration increased(Fig.5)thus indicates that the adsorption amount of HEC on the colloidal surface decreased as NH4OH concentration increased.Since less adsorption of HEC on the colloidal-silica-abrasive surface in the slurry led to less agglomeration of colloidal silica abrasive and more adsorption of HEC onto the silicon-wafer surface,the secondary abrasive size in the slurry decreased(Fig.3)and the surface qualities of the pol-ished silicon wafer,namely,haze,roughness(Fig.1),and number of remaining particles,decreased(Fig.2)as NH4OH concentration increased.ConclusionsThe effect of NH4OH concentration onfinal-touch-polishing per-formance was investigated by using an8-inch stock-polished silicon wafer.The surface qualities,such as haze,roughness,and number of remaining particles on silicon-wafer surface,afterfinal-touch pol-ishing would be determined by the secondary-silica-abrasive size in the slurry and the amount of HEC absorbed on silicon-wafer sur-face.Smaller secondary-abrasive size and more absorption of HEC on the silicon-wafer surface lead to less haze and roughness and fewer remaining particles on the silicon-wafer surface.In addition, the secondary-abrasive size in the slurry and the amount of HEC absorbed on the silicon-wafer surface are determined by the NH4OH concentration of the slurry used forfinal-touch polishing;that is,the secondary-abrasive size in the slurry decreases and the amount of HEC absorbed on the silicon-wafer surface increases as the NH4OH concentration of the slurry increases.Remind that the agglomeration of colloidal silica abrasive is determined by the amount of adsorp-tion site(Si-OH)being decreased with increasing NH4OH concen-tration and the absorption amount of HEC on silicon wafer surface is decided by the suspended HEC concentration in the slurry being increasing with NH4OH concentration.The surface qualities after final-touch polishing,namely,haze,roughness,and number of remaining particles on the silicon-wafer surface,are thus enhanced as the NH4OH concentration of the slurry increases.In conclusion, the proper choice of the NH4OH concentration of the slurry for final-touch polishing is the key chemical-engineering factor for obtaining better surface qualities,namely,haze,roughness,and number of remaining particles on the silicon-wafer surface.AcknowledgmentsAll of the experiments in this paper were supported by the Accel-eration Research Program of the Ministry of Education,Science, and Technology,LG Siltron,Inc.and the Brain Korea21Project in 2010.Hanyang University assisted in meeting the publication costs of this article.References1.Y.Liu,K.Zhang,F.Wang and W.Di,Microelectron.Eng.,66,438(2003).2.S.K.Kim,Y.H.Kim,U.Paik T.Katoh,and J.G.Park,J.Electroceram.,17,835(2006).3.K.C.Cho,H.Jeon,and J.G.Park,J.Korean Phys.Soc.,48,L507(2006).4.H.S.Hwang,J.H.Lim,J.H.Park,E.S.Choi,J.W.Ahn and J.G.Park,Electro-chem.Solid-State Lett.,13,H147(2010).5.The International Technology Roadmap for Semiconductors(2009).6. B.Y.Park,S.H.Jeong,H.S.Lee,H.G.Kim,H.D.Jeong and D.A.Dornfeld,Jpn.J.Appl.Phys.,48,116505(2009).7.H.Yang,X.Song,G.Qiu,M.Tang and S.Yang,J.Electrochem.Soc.,156,H396(2009).8.X.Song,H.Yang,H.Liu,X.Zhang and G.Qiu,J.Electrochem.Soc.,155,D323(2008).9.H.S.Hwang,J.H.Park,S.H.Lee,U.Paik and J.G.Park,Jpn.J.Appl.Phys.,49,010216(2010).10. C.W.Hoogendam,I.Derks,A.de Keizer,M.A.Cohen Stuart and B.H Bijster-bosch,Colloids Surf.,144,245(1998).11.M.Itano,F.W.Kern,R.W.Rosenberg,M.Masayuki,I.Kawanabe and T.Ohmi,IEEE Trans.Semicond.Manuf.,5,114(1992).12.M.Itano,T.Kezuka,M.Ishii,T.Unemoto and M.Kubo,J.Electrochem.Soc.,142,971(1995).13.G.B.Basim and B.M.Moudgil,J.Colloid Interface Sci.,256,137(2002).14.M.Grunder and H.Jacob,Appl.Phys.A,39,73(1986).15.G.M.Grunder and R.Schulz,J.Vac.Sci Technol.,A,7,808(1989).Journal of The Electrochemical Society,158(6)H641-H644(2011) H644。
Substituted donor atoms in silicon crystal for qua
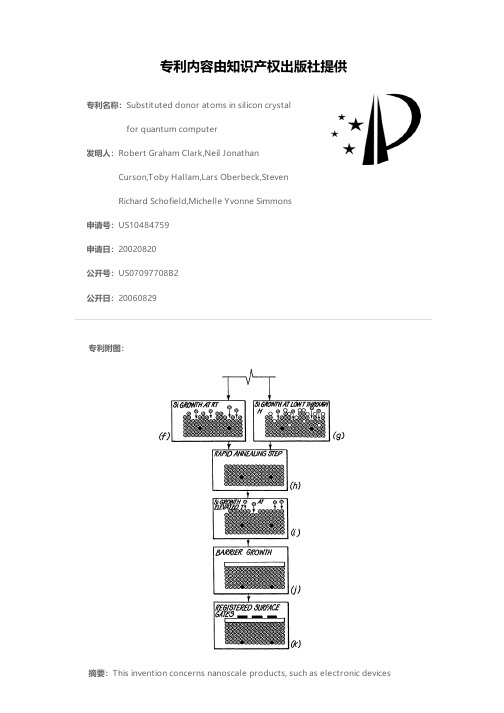
专利名称:Substituted donor atoms in silicon crystalfor quantum computer发明人:Robert Graham Clark,Neil JonathanCurson,Toby Hallam,Lars Oberbeck,StevenRichard Schofield,Michelle Yvonne Simmons申请号:US10484759申请日:20020820公开号:US07097708B2公开日:20060829专利内容由知识产权出版社提供专利附图:摘要:This invention concerns nanoscale products, such as electronic devicesfabricated to nanometer accuracy. It also concerns atomic scale products. These products may have an array of electrically active dopant atoms in a silicon surface, or an encapsulated layer of electrically active donor atoms. In a further aspect the invention concerns a method of fabricating such products. The methods include forming a preselected array of donor atoms incorporated into silicon. Encapsulation by growing silicon over a doped surface, after desorbing the passivating hydrogen. Also, using an STM to view donor atoms on the silicon surface during fabrication of a nanoscale device, and measuring the electrical activity of the donor atoms during fabrication of a nanoscale device. Such products and processes are useful in the fabrication of a quantum computer, but could have many other uses.申请人:Robert Graham Clark,Neil Jonathan Curson,Toby Hallam,LarsOberbeck,Steven Richard Schofield,Michelle Yvonne Simmons地址:Balgowlah Heights AU,Coogee AU,Enmore AU,Coogee AU,Randwick AU,Coogee AU国籍:AU,AU,AU,AU,AU,AU代理机构:Wood, Phillips, Katz, Clark & Mortimer更多信息请下载全文后查看。
二维钙钛矿器件旋涂过程
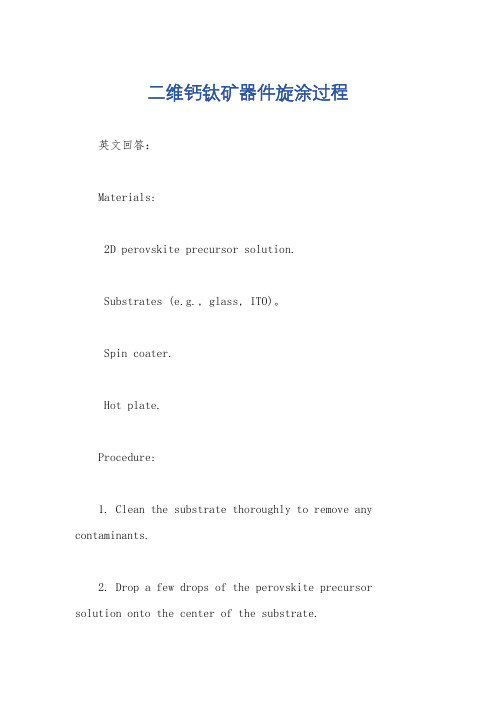
二维钙钛矿器件旋涂过程英文回答:Materials:2D perovskite precursor solution.Substrates (e.g., glass, ITO)。
Spin coater.Hot plate.Procedure:1. Clean the substrate thoroughly to remove any contaminants.2. Drop a few drops of the perovskite precursor solution onto the center of the substrate.3. Spin the substrate at a desired speed andacceleration for a specified time to spread the solution evenly over the surface.4. Ramp down the spinning speed gradually to prevent droplet formation.5. Anneal the spin-coated film on a hot plate at an appropriate temperature to crystallize the perovskite layer.Optimization Parameters:Spin speed: Affects the film thickness and morphology. Higher speeds result in thinner films.Acceleration: Determines the spread rate of thesolution on the substrate.Spinning time: Controls the amount of solution deposited on the substrate.Annealing temperature: Influences the crystal structure and optical properties of the perovskite film.Tips:Use a high-quality perovskite precursor solution to ensure a uniform and stable film.Optimize the spin coating parameters to achieve the desired film thickness and morphology.Control the annealing temperature carefully to avoid degradation of the perovskite layer.中文回答:材料:二维钙钛矿前驱溶液。
- 1、下载文档前请自行甄别文档内容的完整性,平台不提供额外的编辑、内容补充、找答案等附加服务。
- 2、"仅部分预览"的文档,不可在线预览部分如存在完整性等问题,可反馈申请退款(可完整预览的文档不适用该条件!)。
- 3、如文档侵犯您的权益,请联系客服反馈,我们会尽快为您处理(人工客服工作时间:9:00-18:30)。
A quantum
wire effect
School of Engineering, Duke University, Durham, North Carolina 27706
(Received 13 March 1990; acceptedfor publication 25 November 1990) Porous silicon layers grown on nondegenerated p-type silicon electrodesin hydrohuoric acid electrolytes are translucent for visible light, which is equivalent to an increasedband gap compared to bulk silicon. It will be shown that a two-dimensional quantum confinement (quantum wire) in the very narrow walls between the pores not only explains the change in band-gap energy but may also be the key to better understanding the dissolution mechanism that leads to porous silicon formation. The formation of porous silicon layers (PSLs) on silicon electrodesin hydrofluoric acid electrolytes under anodic bias was first described by Uhlir' and Turner.' The properties of porous silicon layers were studied intensively becauseof their potential use in producing silicon layers fully isolated by porous oxidized silicon (FIPOS) .3 The diameter of PSL pores or channels was found to range from 1 up to 100 nm associatedwith porosities of 20-80%. The channels in nondegenerated p-type silicon were found to be the smallest (typically around 2 nm)."' The formation mechanism itself and especially the reason why the walls betweenthe pores are so remarkably resistant against further chemical attack of the electrolyte are still poorly understood. For the smaller ones of the dimensions mentioned above, semiconductors show specific quantum effects due to geometrical constraints7*"In this letter we will show that formation of PSL on nondegenerated p-type silicon is a self-adjusting processdue to a geometrical quantum well or wire effect associatedwith the thin silicon walls remaining between the pores. In our experiments all porous silicon layers were grown in a 1:l (98 wt.% ethanol: 48 wt.% HF) electrolyte using a power supply working as a current source and a Pt working electrode. The sampleswere kept in the dark during PSL formation. In order to measurethe optical absorption of PSL it was necessaryto fabricate layers of about 100 pm separatedfrom the substrate. PSL on single crystalline silicon (5 R cm boron doped, polished surface) was grown in a setup similar to the one used by Turner2 (55 mA/cm2, 45 min). The PSL can be easily separatedfrom the silicon substrate by reducing the electrolyte concentration to 1 wt.% aqueousHF and electropolishing (at 100 mA/cm2 for 10 s). The PSL ( 110pm thickness) has to be kept under water or ethanol becausein air it will easily break, disintegrate into small pieces, and curl up to small loops of approximately 500 pm diameter during drying. Porous silicon layers grown on lightly doped substratesappear to be translucent from dark red to light orange in color after separation from the substrate. The porosity of this layer is high enough (600 m2/cm3)5 to be etched significantly even in pure water (observedby H, evolution). A changein color from red to orange to yellow is observableduring this etching. It was not possibleto separatePSL grown on a degeneratedp + -type silicon sample from the substrate with the electropolishing procedure described above. Therefore a stripe-shapedsilicon sample ( 10 mm x 100 mmx 500 pm, 0.01 fi cm p type) was slightly beveledby grinding (minimum thickness: 109pm, 0.7"beveling angle). The thinned end of the beveledstripe was immersed into a beaker filled with the electrolyte (for 30 min at a current density of 55 mA/cm2). PSL grows on both sides of the stripe. Consequently, the remaining silicon substrate inbetween the growing porous silicon layers becomesthinner and thinner, until it is finally totally transformed into porous silicon. In contrast to PSL on lightly doped substrates, no obvious change in the optical appearance (compared to the substrate itself) was found for PSL grown on the degenerated substrate. The PSL samples ( 110 pm grown on p-type, 150 pm grown on p ' -type substrate) and a lightly doped singlecrystal silicon reference sample (40 pm) were clamped between two 500 [lrn quartz plates under wet conditions and illuminated with monochromatic light (Bausch & Lomb monochromator, 675 grooves/mm) while the intensity of the transmitted light was measured using a germanium detector. The measuredvalues were compared to the ones measuredfor two wet quartz plates only. No corrections were made for the reflections caused by the samples. In order to understand the PSL formation it is necessary to deal with the dissolution chemistry of silicon anodically biased in hydrofluoric acid. A silicon surface is known to be virtually inert against attack of hydrofluoric acid at low pH values, which corresponds to low OH concentrations.'If the silicon is under anodic bias, PSL formation is observedas long as the reaction is limited by the charge supply of the electrode and not by ionic diffusion in the electrolyte. This condition is fulfilled for current densitiesbelow a I3F concentration dependent,critical current density Jpst,." The inner surface of PSL was found to be covered with hydrogen." From these facts and observations of charge transfer ratios at silicon electrodes in hydrofluoric acid, which will be discussed in detail elsewhere,t2 the dissolution mechanism shown in Fig. 1 has been derived. For PSL formation it is essential that a silicon surface saturated by hydrogen is virtually inert against further attack of fluoride ions as long as no electronic holes (h + ) are availableat the silicon electrodebecausethe electronegativity of H is about that of Si and the induced polarization is low. 1:f a hole (h + ) reaches the surface nu-