types_of_nuclear_reactors
常见核能专业英语词汇
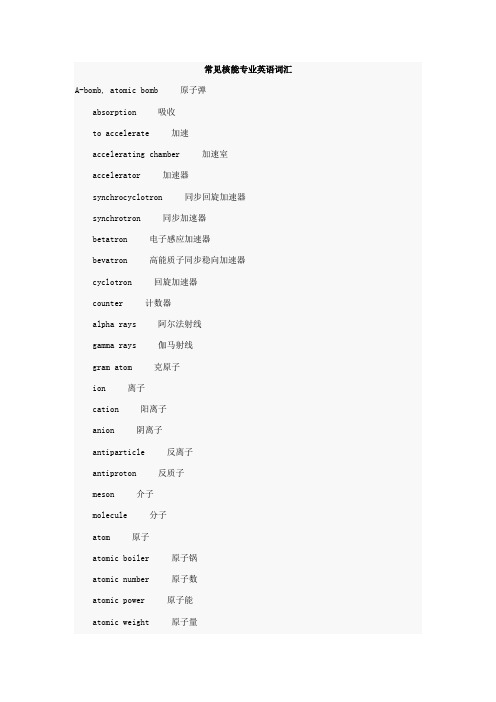
常见核能专业英语词汇A-bomb, atomic bomb 原子弹absorption 吸收to accelerate 加速accelerating chamber 加速室accelerator 加速器synchrocyclotron 同步回旋加速器synchrotron 同步加速器betatron 电子感应加速器bevatron 高能质子同步稳向加速器cyclotron 回旋加速器counter 计数器alpha rays 阿尔法射线gamma rays 伽马射线gram atom 克原子ion 离子cation 阳离子anion 阴离子antiparticle 反离子antiproton 反质子meson 介子molecule 分子atom 原子atomic boiler 原子锅atomic number 原子数atomic power 原子能atomic weight 原子量particle 粒子photon 光子nucleon 核子proton 质子neutron 中子electron 电子free electron 自由电子electron beam 电子束electron cloud 电子云electron gun 电子枪electronic shell 电子层electron volt 电子伏特electrode 电极attraction 引力element 元素barium 钡berkelium 锫beryllium 铍cobalt 钴uranium 铀thorium 钍cadmium 镉caesium, cesium 铯curium 锔radium 镭lithium 锂neptunium 镎strontium 锶boron 硼xenon 氙deuterium 氘krypton 氪deuteron 氘核helium 氦radon 镭射气,氡graphite 石墨heavy water 重水binding energy 结合能blast wave 冲击波bombardment 轰击breeder reactor 增值反应堆bubble chamber 气泡室burst 爆炸,爆发capture 俘获chain reaction 链式反应charge 装燃料cladding 覆层antimatter 反物质clean bomb 干净核弹to collide, collision 碰撞contamination 沾染,污染coolant 冷却剂cooling 冷却cooling fluid 冷却液cooling pond 凉水池core 反应堆心,反应堆活性区cosmic rays 宇宙线critical mass 临界物质curie 居里to decay 衰减decontamination 去污diffusion 漫射disintegration 衰变,蜕变dispersion 弥散emission 发射enriched uranium 浓缩铀enrichment 浓缩explosion 爆炸,爆裂fertile element 可转换元素fission 裂变fissionable material 裂变物质fusion 聚变deflagration 爆燃过程detector 探测器half-life 半衰期H-bomb, hydrogen bomb 氢弹heat exchanger 热交换器heterogeneous reactor 非均匀反应堆homogeneous reactor 均匀反应堆instability 不稳定性ionization 电离作用irradiation 照射,辐射isomer 同质异能素isotope 同位素kiloton 千吨当量labelled molecule, tagged molecule 标记分子leakage 泄漏lifetime 寿命mass 质量megaton 百万吨级moderator 减速剂mushroom cloud 蘑菇云neutron flux 中子通量nucleus 核nuclear tests 核实验nuclear physics 核物理nuclear power plant, nuclear power station 核电站nuclear reactor 核反应堆orbital electron, planetary electron 轨道电子power reactor 动力反应堆projectile 抛射体quantum number 量子数radiant energy 辐射能radiation 辐射radioactive cloud 放射云radioactive elements 放射性元素radioactive fallout 放射性尘降物radioactivity 放射性radioisotope 放射性同位素radiology 放射学radiotherapy 放射疗法rod 棒roentgen 伦琴scattering 散射separation 分离shield 防护罩shock wave 冲击波spectrometer 分光计spin 反旋to split 分裂stability 稳定,对衰变的抗性thermal neutron 热中子thermal reactor 热反应堆thermionic 热电子的thermonuclear 热核的tracer element 示踪原子trajectory 轨迹trinitrotoluene 三硝基甲苯,黄色炸药underwater test 水下试验warhead 弹头。
IEEEStdC57.124-1991

IEEE Std C57.124-1991Reconized as an American National Standard (ANSI)IEEE Recommended Practice for theDetection of Partial Discharge and the Measurement of Apparent Charge inDry-Type TransformersSponsorTransformers Committeeof theIEEE Power Engineering SocietyApproved June 27, 1991Reaffirmed February 6, 1997Institute of Electrical and Electronics EngineersApproved October 11, 1991Reaffirmed September 19, 1996American National Standards InstituteAbstract: IEEE Std C57.124-1991 covers the detection of partial discharges occurring in the insulation of dry-type transformers of their components and the measurement of the associated apparent charge at the terminals when alternating test voltage is applied. The wideband method is used. The detection system and calibrator characteristics are described, and the test procedure is established.Keywords: Apparent charge, corona, cost coil transformers, dry-type transformers, partial discharge, ventilated dry-type transformersThe Institute of Electrical and Electronics Engineers, Inc.345 East 47th Street, New York, NY 10017-2394, USACopyright © 1992 byThe Institute of Electrical and Electronics Engineers, Inc.All rights reserved. Published 1992Printed in the United States of AmericaISBN 1-55937-159-5No part of this publication may be reproduced in any form, in an electronic retrieval system or otherwise, without the prior written permission of the publisher.IEEE Standards documents are developed within the Technical Committees of the IEEE Societies and the Standards Coordinating Committees of the IEEE Standards Board. Members of the committees serve voluntarily and without compensation. They are not necessarily members of the Institute. The standards developed within IEEE represent a consensus of the broad expertise on the subject within the Institute as well as those activities outside of IEEE that have expressed an interest in participating in the development of the standard.Use of an IEEE Standard is wholly voluntary. The existence of an IEEE Standard does not imply that there are no other ways to produce, test, measure, purchase, market, or provide other goods and services related to the scope of the IEEE Standard. Furthermore, the viewpoint expressed at the time a standard is approved and issued is subject to change brought about through developments in the state of the art and comments received from users of the standard. Every IEEE Standard is subjected to review at least every five years for revision or reaffirmation. When a document is more than five years old and has not been reaffirmed, it is reasonable to conclude that its contents, although still of some value, do not wholly reflect the present state of the art. Users are cautioned to check to determine that they have the latest edition of any IEEE Standard.Comments for revision of IEEE Standards are welcome from any interested party, regardless of membership affiliation with IEEE. Suggestions for changes in documents should be in the form of a proposed change of text, together with appropriate supporting comments.Interpretations: Occasionally questions may arise regarding the meaning of portions of standards as they relate to specific applications. When the need for interpretations is brought to the attention of IEEE, the Institute will initiate action to prepare appropriate responses. Since IEEE Standards represent a consensus of all concerned interests, it is important to ensure that any interpretation has also received the concurrence of a balance of interests. For this reason IEEE and the members of its technical committees are not able to provide an instant response to interpretation requests except in those cases where the matter has previously received formal consideration.Comments on standards and requests for interpretations should be addressed to:Secretary, IEEE Standards Board445 Hoes LaneP.O. Box 1331Piscataway, NJ 08855-1331USAIEEE Standards documents are adopted by the Institute of Electrical and Electronics Engineers without regard to whether their adoption may involve patents on articles, materials, or processes. Such adoption does not assume any liability to any patent owner, nor does it assume any obligation whatever to parties adopting the standards documents.Foreword(This foreword is not a part of IEEE Std C57.124-1991, IEEE Recommended Practice for the Detection of Partial Discharge and the Measurement of Apparent Charge in Dry-Type Transformers.)This recommended practice for measuring partial discharge of dry-type transformers was conceived for the purpose of establishing a standardized method for conducting partial discharge tests of dry-type transformers. The results of the tests may be compared with various transformer designs and manufacturers to establish a partial discharge limit for dry-type transformers.This recommended practice follows the format of IEEE Std 454, IEEE Recommended Practice for the Detection and Measurement of Partial Discharge (Corona) During Dielectric Tests, and IEEE Std C57.113, IEEE Guide for Partial Discharge Measurement in Liquid-Filled Power Transformers and Shunt Reactors. Sections on current detection were purposely omitted, as this technique is normally not used for dry-type transformers.There is no recognized definition of “partial discharge-free"”when referring to partial discharge inception voltage or extinction voltage. An arbitrary sensitivity of 10 pC is suggested until such time as a more definitive standard is established.Various specifications are already written specifying partial discharge-free transformers from 1.1 p.u. operating voltage to 2.0 p.u. operating voltage. It is the intent of this recommended practice to encourage manufacturers of dry-type transformers and users of dry-type transformers to investigate and report the results of factory tests and field experience of partial discharge in dry-type transformers. It is recognized that Paschen's Law applies to the partial discharge intensity and extinction voltage of dry-type transformers. It is conceivable that a dry-type transformer would test partial discharge-free at 1.65 p.u. voltage at room temperature and be barely partial discharge-free at operating temperature for a Class 220C. system. This correlation should be verified with field experience and reported.The guide specifies no particular discharge testing instruments and systems. Several commercially available units are being used. A measuring system of discreet components readily available has been used for measuring partial discharge. Most manufacturers' laboratories have partial discharge-free HV test sets and oscilloscopes. The only additional components required to complete the detection circuit are a partial discharge-free coupling capacitor composed of two 60 kV, .002 mfd capacitors in series, and an inductance composed of a coil of magnet wire. Calibration is accomplished using a calibrated square wave generator and a calibrated coupling capacitor of .0001 mfd.The following two test procedures are proposed:1)To test partial discharge between the coil and ground, normally accomplished during the applied voltage test.2)The test procedure takes place during the induced voltage test to detect partial discharge within a coil. It issuggested that partial discharge measurements be made in both modes. The partial discharge measurement may be made during the normal sequence of tests, while the applied and induced voltage tests are being made.An alternative sequence is to conduct the partial discharge test immediately following the applied voltage test and induced voltage test.The high-voltage bus bars of high-voltage transformers sometimes cause nondestructive partial discharge. This partial discharge in no way affects the reliability of the transformer coils. It may be necessary to disconnect the bus bar from the coils before conducting the partial discharge test on only the coils in order to test for partial discharge in the transformer coils. A note should be added to any test reports stating that the bus bar was removed for the test.At the time this document was submitted to the Standards Board, the Working Group on Recommended Practice for the Detection of Partial Discharges and the Measurement of Apparent Charge in Dry-Type Transformers had the following members:A.D. Kline, (Chairman)B. F. Allen Roy Bancroft D. A. Barnard A. Bimbiris M. Cambre O. R. Compton J. FrankE. Gearhart R. Hayes R. H. HollisterJ. W. HuppA.M. IversenA. J. JonnattiS. P. KennedyE. KoenigM. L. ManningR. A. MarekM. I. MitelmanJ. J. NayW. F. PattersonR. L. ProvostJ. RoddenV. ThenappanR. E. Uptegraff, Jr.G. H. VaillancourtH. J. WindischThe following persons were on the balloting committee that approved this document for submission to the IEEE Standards Board:E. J. Adolphsen L. C. Aicher D. J. AllanB. AllenR. Allustriarti M. S. Altman J. C. Arnold J. AubinR. Bancroft D. Barnard D. L. Basel P. L. Bellaschi S. Bennon W. B. Binder J. V. Bonucchi J. D. Borst C. V. Brown O. R. Compton F. W. Cook J. L. Corkran D. W. Crofts J. N. Davis D. J. Douglas R. F. Dudley J. C. Dutton J. K. Easley J. A. Ebert D. J. Fallon F. L. Foster M. Frydman H. E. Gabel R. E. Gearhart D. W. Gerlach D. A. Gillies R. S. Girgis R. L. GrubbF. J. GryszkiewiczG. HallJ. H. HarlowF. W. HeinrichsW. R. HenningD. R. HightonP. J. HoeflerC. HoeselR. H. HollisterC. C. HoneyE. HowellsC. HurryG. W. IliffY. P. IijimaR. G. JacobsenD.C. JohnsonD. L. JohnsonA. J. JonnattiC. P. KappelerR. B. KaufmanJ. J. KellyW. N. KennedyJ.P. KinneyB. KlaponskiA.D. KlineE. KoenigJ. G. LackeyR. E. LeeH.F. LightS.R. LindgrenL.W. LongL. A. LowdermilkR. I. LoweM. L. ManningH. B. MargolisT. MassoudaJ. W. MatthewsJ. McGillC. J. McMillenW. J. McNuttS. P. MehtaC. K. MillerC. H. MillianR. E. MinkwitzM. MitelmanH. R. MooreW. E. MorehartR. J. MuselW. H. MutschlerE. T. NortonR. A. OlssonB. K. PatelW. F. PattersonH. A. PearceD. PercoL. W. PierceJ. M. PollittC. P. RaymondC. A. RobbinsL. J. SavioW. E. SaxonD. N, SharmaV. ShenoyW. W. SteinL. R. StenslandD. SundinL. A. SwensonD. S. TakachV. ThenappanR. C. Thomas J. A. Thompson T. P. Traub D. E. Truax W. B. Uhl R. E. Uptegraff, Jr.G. H. VaillancourtA. VeitchL. B. WagenaarR. J. WheartyA. L. WilksW. E. WrennA. C. WurdackE. J. YasudaAt the time this recommended practice was published, it was under consideration for approval as an American National Standard. The Accredited Standards Committee on Transformers, Regulators, and Reactors, C57, had the following members at the time this document was sent to letter ballot:Leo J. Savio, ChairJohn A. Gauthier, SecretaryOrganization of Representative Electric Light and Power Group...............................................................................................P.E. OrehekS. M. A. RizviF. StevensJ. SullivanJ. C. ThompsonM.C. Mingoia (Alt.) Institute of Electrical and Electronics Engineers......................................................................J. D. BorstJ. DavisJ. H. HarlowL. SavioH. D. SmithR. A. VeitchNational Electrical Manufacturers Association........................................................................G. D. CoulterP. DeweverJ. D. DouglasA. A. GhafourianK. R. LinsleyR. L. PlasterH. RobinR. E. Uptegraff, Jr.P. J. Hopkinson (Alt.)J. Nay (Alt.) Tennessee Valley Authority.......................................................................................................F. A. Lewis Underwriters Laboratories, Inc.................................................................................................W. T. O'GradyUS Department of Agriculture, REA........................................................................................J. BohlkUS Department of Energy, Western Area Power Administration.............................................D. R. TorgersonUS Department of the Interior, Bureau of Reclamation............................................................F. W. Cook, Sr.US Department of the Navy, Civil Engineering Carps.............................................................H. P. StickleyWhen the IEEE Standards Board approved this standard on June 27, 1991, it had the following membership:Marco W. Migliaro, ChairDonald C. Loughry, Vice ChairAndrew G. Salem, SecretaryDennis BodsonPaul L. BorrillClyde CampJames M. Daly Donald C. Fleckenstein Jay Forster*David F. Franklin Ingrid Fromm Thomas L. HannanDonald N. HeirmanKenneth D. HendrixJohn W. HorchBen C. JohnsonIvor N. KnightJoseph Koepfinger*Irving KolodnyMichael A. LawlerJohn E. May, Jr.Lawrence V. McCAllT. Don Michael*Stig L. NilssonJohn L. RankineRonald H. ReimerGary S. RobinsonTerrance R. Whittemore*Member EmeritusAlso included are the following nonvoting IEEE Standards Board liaisons:Fernando Aldana Satish K. AggarwalJames Beall Richard B. EngelmanStanley Warshaw Deborah A. CzyzIEEE Standards Project EditorCLAUSE PAGE1. Scope (1)2. Purpose (1)3. References (1)4. Definitions (2)5. Partial Discharge Detection System (3)5.1High-Voltage Coupling Circuit (3)5.2Measuring Impedance Unit (Z m) (4)5.3Filter Characteristics (5)5.4Display Unit (5)5.5Discharge Meter (6)5.6Basic Sensitivity (6)5.7Partial Discharge Detector Basic Sensitivity Test (6)6. Calibrator Characteristics (6)6.1Calibrating Capacitor Value (C q) (7)6.2Pulse Generator Rise Time and Decay Time (7)6.3Pulse Generator Amplitude (U o) (7)6.4Pulse Generator Output Impedance (Z o) (7)6.5Calibrator Output Level Adjustment (7)6.6Pulse Generator Frequency (7)7. Tests (7)7.1General Requirements (7)7.2Conditioning (8)7.3Requirements for the Test Voltage (8)7.4Transformer Connections (8)7.5Significance of Various Test Connections (8)7.6Choice of Test Procedure (9)7.7Disturbances (10)8. Bibliography (18)Annex (informative) Partial Discharge Recognition (24)IEEE Recommended Practice for the Detection and the Measurement of Partial Discharge in Dry-Type Transformers1. ScopeThis recommended practice applies to the detection of partial discharges occurring in the insulation of dry-type transformers or their components, and to the measurement of the associated apparent charge at the terminals when an alternating test voltage is applied.2. PurposePartial discharge measurements in dry-type transformers may preferably be made on the basis of measurement of the apparent charge. Relevant measuring systems are classified as narrow-band or wide-band systems. Both systems are recognized and widely used. Without giving preference to one or the other, it is the object of this document to describe the wide-band method. General principles of partial discharge measurements, including the narrow-band method, are covered in IEEE Std 454-19731 [8]2, IEC 270 (1981) [6]3and IEC 76-3 (1980) [5].3. ReferencesThe following publications should be used in conjunction with this document. When the standards referred to in this guide are superseded by a new revision approved by the relevant standards authority, the latest revision should apply.[1] ANSI C68.1-1968, Standard for Measurement of Voltage In Dielectric Tests.41This standard has been withdrawn, however, copies are available from the Institute of Electrical and Electronics Engineers, Inc., Service Center, 445 Hoes Lane, Piscataway, N.J. 08855, U.S.A.2The numbers in brackets refer to those listed in Section 4.3IEC publications are available from IEC Sales Department, Case Postale 131, 3 rue de Varembé, CH 1211, Genève 20, Switzerland/Suisse. IEC publications are also available in the United States from the Sales Department, American National Standards Institute, 11 West 42nd Street, 13th Floor, New York, NY 10036, USA.4ANSI publications are available from the American National Standards Institute, 11 West 42nd Street, 13th Floor, New York, NY 10036.IEEE Std C57.124-1991IEEE RECOMMENDED PRACTICE FOR THE DETECTION OF PARTIAL DISCHARGE [2] ASTM D1868-81 (1990-E01), Method for Detection and Measurement of Partial Discharge (Corona) Pulses in Evaluation of Insulation Systems.5[3] ASTM STP-669, Engineering Dielectrics, Vol. 1, Corona Measurement and Interpretation.[4] CIGRE Working Group 21-03, "Recognition of Discharges," Electra, No. 11, pp. 61-98, Dec, 1969.6[5] IEC 76-3 (1980), Power Transformers, Part 3: Insulation Levels and Dielectric Tests.7[6] IEC 270 (1981), Partial Discharge Measurements.[7] IEEE Std 436-1991, IEEE Guide for Making Corona (Partial Discharge) Measurements of Electronics Transformers (ANSI).8[8] IEEE Std 454-1973, IEEE Recommended Practice for the Detection and Measurement of Partial Discharge (Corona) During Dielectric Tests.[9] IEEE Std C57.113-1991, IEEE Guide for Partial Discharge Measurement in Oil-Filled Power Transformers and Shunt Reactors (ANSI).4. Definitionspartial discharge: A partial discharge within the terms of this document is an electric discharge that only partially bridges the insulation between conductors. The term “corona ” has also been used frequently with this connotation. Such usage is imprecise and is gradually being discontinued in favor of the term “partial discharge.”apparent charge (terminal charge): The apparent charge (q) of a partial discharge is that charge which, if it could be injected instantaneously between the terminals of the test object, would momentarily change the voltage between its terminals by the same amount as the partial discharge itself. The apparent charge should not be confused with the charge transferred across the discharging cavity in the dielectric medium. Apparent charge within the terms of this document is expressed in coulombs, abbreviated C. One pC is equal to 10-12 coulombs.repetition rate(n).: The partial discharge pulse repetition rate (n) is the average number of partial discharge pulses per second measured over a selected period of time.acceptable terminal partial discharge level: The acceptable terminal partial discharge level is that specified maximum terminal partial discharge value for which measured terminal partial discharge values exceeding the said value are considered unacceptable. The method of measurement and the test voltage for a given test object should be specified with the acceptable terminal partial discharge level.voltage related to partial discharges: Voltage within the terms of this document is the phase-to-ground alternating voltage for applied tests (Fig 1) or terminal to terminal alternating voltage for induced voltage tests (Fig 8). Its value is expressed by its peak value divided by the square root of two.partial discharge inception voltage: The lowest voltage at which partial discharges exceeding a specified level are observed under specified conditions when the voltage applied to the test object is gradually increased from a lower value. This voltage is expressed as the peak value divided by the square root of two.5ASTM publications are available from the American Society for Testing and Materials, Customer Service Dept., 916 Race Street, Philadelphia, P.A. 19103, U.S. A.6CIGRE publications are available from the International Conference on Large-Voltage Electric Systems, 112 Boulevard Haussman, F-75008 Paris, France.7IEC publications are available from IEC Sales Department, Case Postale 131, 3 rue de Varembé, CH 1211, Genève 20, Switzerland/Suisse. IEC publications are also available in the United States from the Sales Department, American National Standards Institute, 11 West 42nd Street, 13th Floor, New York, NY 10036, USA.8IEEE publications are available from the Institute of Electrical and Electronics Engineers, Inc., Service Center, 445 Hoes Lane, Piscataway, N.J. 08855, U.S. A.partial discharge extinction voltage: The voltage at which partial discharges exceeding a specified level cease under specified conditions when the voltage is gradually decreased from a value exceeding the inception voltage. This voltage is expressed as the peak value divided by the square root of two.partial discharge-free test voltage: The partial discharge-free test voltage is a specified voltage, applied in accordance with a specified test procedure, at which the test object should not exhibit partial discharges above the acceptable energized background noise level.energized background noise level: The energized background noise level stated in pC is the residual response of the partial discharge measurement system to background noise of any nature after the test circuit has been calibrated and the test object is energized at a maximum of 50% of its nominal operating voltage.acceptable energized background noise level: The acceptable energized background noise level present during test should not exceed 50% of the acceptable terminal discharge level, and in any case, should be below 100 pC (5 pC if an acceptable terminal discharge level of 10 pC is required.)5. Partial Discharge Detection System(Figs 9 and 10 taken from ASTM STP669)The partial discharge detection system comprises the following components:1) a high-voltage coupling circuit (C1, C v)2) a measuring impedance unit (Z m consisting of R m, C2 and L)3)an amplifier and filter circuit4) a display unit5) a discharge meter6) a calibrator (C q, V l)7) a source filter (Z optional)5.1 High-Voltage Coupling CircuitThe purpose of the high-voltage coupling circuit is to allow the connection of the measuring impedance (Z m) to the high-voltage terminal of the transformer under test. In other types of high-voltage equipment, a single, low-capacitance high-voltage capacitor is usually used for this purpose, but in transformers, the equivalent terminal capacitance is usually very low, so a substantial amount of signal is normally produced by partial discharge of only a few pC, and measurement sensitivity is not a problem. At the same time, due to standing waves within the winding, a certain amount of signal cancellation may occur if the bandwidth is not sufficiently wide. Therefore, to insure that the input circuit of the partial discharge detection system does not act as a differentiator and reduce the total bandwidth of the system, it has been found that a high value for the coupling capacitor is necessary to produce a long-time constant of the input circuit. Even then, however, the low equivalent terminal capacitance of the transformer, usually less than 500 pF, will limit this time constant and it may not be possible to make it sufficiently long. Therefore, the use of a single coupling capacitor is not recommended. On the other hand, a satisfactory time constant can always be obtained by using a second capacitor (C2) as part of the measurement impedance, and this is the recommended method.As shown in Figs 9 and 10, high-voltage capacitor C1, and low-voltage capacitor C2, will form a voltage divider. This voltage divider will reduce the sensitivity of the measurement. To make sure that both the sensitivity is still sufficient and the time constant is sufficiently long, the values of C1, C2, and L should respect the conditions below:(1)≥C1100pF(2)where R m is the parallel resistive part of the measuring impedance unit (Z m ). The value of R m should be determined from the particular partial discharge instrument that is used.Example:For f L = 70kHz ,R m = 2.5k Ω and C 1 = 100pFA value of 1000 pF may be chosen for C 2 since 1000 pF ≥ 909 pF and (3)In cases where an RLC measurement impedance is used, the value of L should satisfy the equation below. This will ensure that the measurement bandwidth is unaffected by the presence of L .(4)If the transformer to be tested is fitted with a capacitive bushing tap, then this can be used directly as the high-voltage coupling circuit, and a separate coupling capacitor C 1 is not needed.5.2 Measuring Impedance Unit (Z m )The measuring impedance unit (Z m ) is located physically close to the high-voltage coupling circuit and serves two main purposes:1)It attenuates the test voltage present on the high-voltage coupling circuit to a safe value for measurement of partial discharge signals;2)It matches the amplifier and filter circuit to the high-voltage coupling circuit in insuring a fiat frequencyresponse across the full measurement bandwidth.The measuring impedance unit (Z m )should be configured in such a way as to permit test voltage level monitoring and to observe the phase relationship between the test voltage and the partial discharge pulses; this technique helps to identify the nature of the discharges.As shown in Figs 9 and 10, capacitor C v , whose capacitance value should be chosen to be at least 500 times that of C 1,may be placed in either one of the following two positions:1)In series with inductor L (Fig 9 or 10), or 2)In series with the low-voltage side of high-voltage capacitor C 1 (Fig 10.)C 212llf L R m -------------------≥C 216.28x 70000x 2500,----------------------------------------------909pF ==1000100-----------1015≤=L 25006.28x 70000,------------------------------- 5.7mH ==C 2C 1------15≤L R m 2llf L -----------≥Figure 9 shows the preferred position for C v since the input impedance of the display unit does not shunt the measurement impedance and can be neglected. However, some voltage will reach the input of the amplifier and filter circuit and may cause it to saturate. This voltage can be decreased by increasing the value of C v until it is less than 5V . If saturation occurs, a 20 nF low-voltage capacitor may be placed in series with the input of the amplifier and filter circuit as shown to decouple voltage at the excitation frequency.In the cases where one can not be absolutely certain that saturation of the amplifier will not occur, it is then advised to place C v in the position shown in Fig 10. The impedance of the display circuit will now shunt the measurement impedance and its input capacitance will need to be considered to calculate the value of C 2, as it will add to it.5.2.1 Lower and Upper Cut-Off Frequencies (f L and f H )The lower and upper cut-off frequencies f L and f H , respectively, are the frequencies at which the response to a constant sinesoidal input voltage has fallen by 6 db from the maximum value occurring inside the recommended bandwidth.f L should be located in the range from 70 to 120 kHz to minimize the effect of winding attenuation on partial discharge signals, and at the same time, to provide adequate rejection of SCR-generated noise present in manufacturing plants.An upper limit on f H of 300 kHz is usually necessary to prevent broadcast stations from interfering with the partial discharge measurement.5.3 Filter CharacteristicsThe filter characteristics of the partial discharge detection circuit should be such as to provide attenuation of at least 50db at 25 kHz, of at least 60 db at 15 kHz and below, and of at least 20 db at 500 kHz and above, with respect to the response at the geometric mean frequency (f C ) of the system pass bandwidth that is given by:The filter may be combined with an amplifier to form an active filter. Care should be taken to prevent the saturation of the filter input by the presence of the applied test voltage.5.3.1 Bandwidth ∆fThe bandwidth ∆f is defined as:∆f = f H − f LThe bandwidth should not be less than 100 kHz. A wider bandwidth provides a response whose level is less sensitive to the location of a partial discharge pulse along a transformer winding and is, therefore, more uniform. A bandwidth wider than 100 kHz is preferable, but may lead to background noise problems.5.3.2 LinearityThe instrument circuit, display unit, and discharge meter should be linear within plus or minus 10% of full scale in the range of 50 to 1000 pC.5.4 Display UnitThe display unit should be a cathode ray oscilloscope with a linear, rectangular, or an elliptical time-base. In all cases,the time-base should be synchronized to the test voltage, and at least 98% of a full cycle should be displayed. The phase relationship of the partial discharges to the test voltage should be easy to determine. A suitable graticule should be provided.f c f L f H •().5=。
核能课件ppt英文
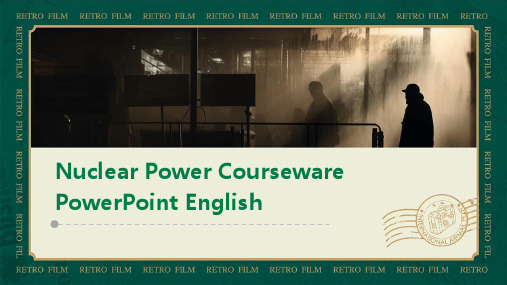
Advantages and Disadvantages of Nuclear Energy
Advantages
Nuclear energy is a sustainable source of energy that can provide a stable and related supply of electricity It does not emit greenhouse gases or air pollutants during operation, making it environmentally friendly Nuclear energy can also be used to produce medical isotopes and for other industrial applications
History of Nuclear Energy
The discovery of nuclear energy Began in the early 20th century with the discovery of radioactivity by Antoine Henri Becquerel in 1896
Nuclear Power Courseware PowerPoint English
• Introduction • Nuclear Physics • Nuclear Reactors • Safety and Security of Nuclear
Reactors • Future of Nuclear Energy
01 Introduction
Definition of Nuclear Energy
核电英语
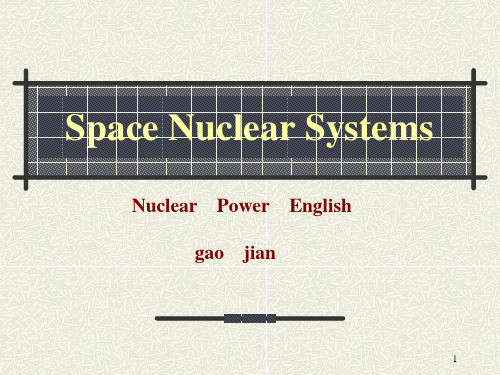
SNAP-10A system
3
Historical
Review
The United States has not launched another reactor system since SNAP-10A; however, the SNAP program developed and ground-tested five space power reactor during the 1960s. One of the reactors, SNAP-8A, was designed to produce 30kW of electrical power. Other space power reactor concepts were explored between the late 1950s and the early 1970s
9
Nuclear Propulsion Systems
The crew and the nuclear propulsion systems are first placed into Earth orbit by conventional launch vehicles. The high velocity nuclear propulsion system is then used to transport the crew to Mars. Shorter trip times, made possible by nuclear propulsion, reduce crew exposure to cosmic radiation and reduce life support system requirements.
4
核电专业英语学习(必考)解析
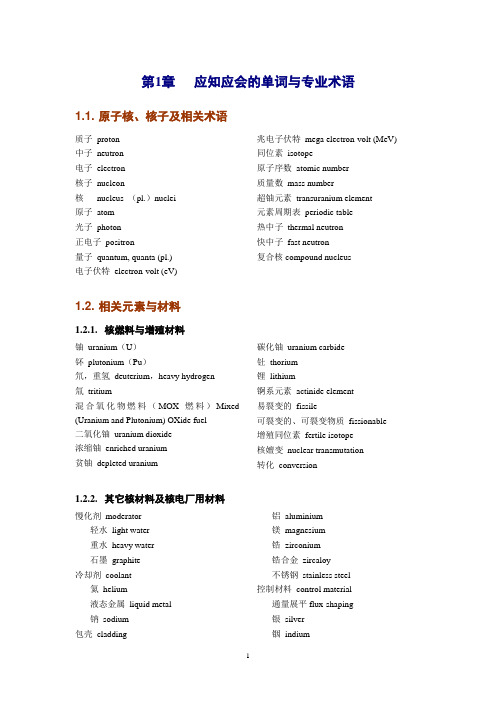
第1章应知应会的单词与专业术语1.1. 原子核、核子及相关术语质子proton中子neutron电子electron核子nucleon核nucleus (pl.)nuclei 原子atom光子photon正电子positron量子quantum, quanta (pl.) 电子伏特electron-volt (eV) 兆电子伏特mega electron-volt (MeV) 同位素isotope原子序数atomic number质量数mass number超铀元素transuranium element元素周期表periodic table热中子thermal neutron快中子fast neutron复合核compound nucleus1.2. 相关元素与材料1.2.1.核燃料与增殖材料铀uranium(U)钚plutonium(Pu)氘,重氢deuterium,heavy hydrogen氚tritium混合氧化物燃料(MOX燃料)Mixed (Uranium and Plutonium) OXide fuel二氧化铀uranium dioxide浓缩铀enriched uranium贫铀depleted uranium 碳化铀uranium carbide钍thorium锂lithium锕系元素actinide element易裂变的fissile可裂变的、可裂变物质fissionable 增殖同位素fertile isotope核嬗变nuclear transmutation转化conversion1.2.2.其它核材料及核电厂用材料慢化剂moderator轻水light water重水heavy water石墨graphite冷却剂coolant氦helium液态金属liquid metal钠sodium包壳cladding铝aluminium镁magnesium锆zirconium锆合金zircaloy不锈钢stainless steel 控制材料control material 通量展平flux-shaping银silver铟indium镉cadmium可燃毒物burnable poison 硼boron硼酸boric acid锂lithium铍beryllium 乏燃料spent fuel因科镍,因康INCONEL不锈钢stainless steel奥氏体不锈钢austenitic stainless steel 铁素体不锈钢ferritic stainless steel马氏体不锈钢martensitic stainless steel1.3. 核反应及相关术语衰变decay裂变fission聚变fusion核反应nuclear reaction链式反应chain reaction截面cross section微观截面microscopic cross section 宏观截面macroscopic cross section 吸收截面absorbing cross section 散射截面scattering cross section靶恩barn缓发中子delayed neutron瞬发中子prompt neutron瞬发临界prompt criticality易裂变的fissile可裂变的fissionable慢化morderate / slow down增殖比breeding ratio燃耗burnup反应性reactivity中子循环neutron cycle裂变产物fission product临界criticality瞬发临界prompt critical通量flux氙xenon 碘iodine锕系(元素)actinide反应性价值reactivity worth慢化剂温度系数 moderator temperature coefficient反应性系数 reactivity coefficient剩余反应性 excess reactivity燃料比功率 fuel specific power倍增因子multiplication factor有效增殖系数effective multiplication factor;effective multiplication constant无限介质增殖系数infinite multiplication factor;infinite multiplication constant快中子增殖系数fast fission factor热中子利用系数thermal utilization factor不泄漏几率nonleakage probability逃脱共振俘获几率resonance escape probability四因子公式four-factor formula多普勒增宽Doppler broadening*总集成中子通量/总积分中子通量Total Integrated Neutron Flux = Integrated Flux or Fluence (注量) = Neutron density ⨯ Velocity ⨯Time [单位:n/m3⋅ m/s ⋅ s = n/m2]1.4. 反应堆压水堆Pressurized Water Reactor (PWR)沸水堆Boiling Water Reactor (BWR)加拿大重水铀反应堆(坎杜堆)CANadian Deuterium and Uranium reactor (CANDU) / pressurized heavy water reactor (PHWR)英国气冷堆(美诺克斯堆)British gas-cooled Magnox reactor高温气冷堆high temperature gas-cooled reactor (HTGR)快中子增殖反应堆fast breeder reactor (FBR)轻水堆Light Water Reactor (LWR)先进反应堆advanced reactor超临界水反应堆supercritical water reactor欧洲压水堆(第三代反应堆之一)European Pressurized water Reactor (EPR)(美国)先进压水堆600/1000(第三代(+)反应堆之一)AP(WR)600 / 10001.5. 核电厂部件、设备与系统燃料芯块fuel pellet燃料元件fuel element燃料棒fuel rod燃料组件fuel assembly定位格架spacer grid法兰flange密封环seal ring阻力塞plug(上/下)腔室(upper / lower ) plenum堆芯,活性区core反应堆压力容器Reactor Pressure V essel (RPV)上封头upper closure head液压螺栓拉伸机(张紧机) hydraulic stud tensioner包覆层clad (碳钢表面的防腐蚀堆焊层) 控制棒control rod控制棒组件Control Element Assembly (CEA)可燃吸收棒burnable absorber rod控制棒驱动机构Control Element Drive Mechanism (CEDM)变送器transmitter信号调理signal regulation吊篮barrel进/出口接管inlet / outlet nozzle 冷/热端,冷/热腿,冷/热管段cold / hot leg 反应堆堆内构件reactor vessel internals肿胀swelling腐蚀corrode, corrosion侵蚀erode, erosion氧化oxidation, oxidize完整性integrity反应堆冷却剂泵(主泵)Reactor Coolant Pump (RCP)屏蔽泵canned (motor) pump轴封泵shaft seal pump反应堆冷却剂系统(一回路系统)Reactor Coolant System (RCS)核蒸汽供应系统Nuclear Steam Supply System (NSSS)一回路primary loop/circuit一回路系统/主回路系统primary system二回路secondary loop稳压器pressurizer (PRZ)波动管surge line汽水分离器moisture separator干燥器(二/三级汽水分离器)steam dryer 安全阀safety valve卸压阀relief valve溢流阀overflow valve主蒸汽隔离阀main steam isolation valve单向阀check valve止回阀non-return valve主蒸汽联箱main steam header给水调节阀feed regulating valve蒸汽发生器Steam Generator (SG)主蒸汽管Main Steam Line (MSL)汽轮机steam turbine汽水分离再热器Moisture Separator Reheater (MSR)给水泵feed (water) pump上充泵charging pump凝汽器condenser发电机(electric) generator安全壳containment地基,基础foundation烟道stack贯穿件penetration核岛nuclear island常规岛conventional island核电厂配套子项Balance of Plant (BOP)一回路辅助系统auxiliary system for primary loop化学与容积控制系统(化容系统)Chemical and V olume Control System (CVCS)专设安全设施Engineered Safety Feature (ESF)余热排出系统Residual Heat-Removal System (RHRS)应急堆芯冷却系统Emergency Core Cooling System (ECCS),安注系统Safety Injection (SI) System直接注射系统direct vessel injection (DVI) 换料水箱In-containment Refueling Water Storage Tank (IRWST) (乏)燃料贮存水池(spent) fuel storage pool 燃料装卸系统fuel handling system堆芯补水箱core makeup tank蓄压箱accumulator机组unit辅助喷淋auxiliary spray柴油发电机Diesel generator自动保护系统Automatic Protective System (APS)自动降压系统automatic depressurization system (ADS)仪控系统Instrumentation and Control System (I & C system)开关设备,开关柜switch gear蒸汽轴封系统,压盖蒸汽密封系统gland steam system汽轮机旁路管turbine bypass line辅助给水泵auxiliary feedwater pump汽动给水泵turbine driven feedwater pump 导管conduit冷凝水泵condensate pump冷凝水增压泵condensate booster pump水润滑轴承water lubricated bearing人孔man way检修孔accessory port热电偶thermocouple加热节点热电偶heated junction thermocouple干簧管开关reed switch流量限制器flow restrictor节流孔版orifice1.6. 反应堆运行运行operation运行工况operating condition 操纵员operator 维护maintenance监督、监视surveillance监督试样surveillance specimen辐照监督管irradiation surveillance capsule 辐照监督试样盒surveillance specimen compartment硼浓度boron concentration稀释dilution / dilute硼注入 boron injection停堆shutdown紧急停堆scram / trip停役outage换料停堆refueling outage / refueling shutdown换料refuel卸料 discharge倒料 shuffling满功率运行full power operation负荷跟踪load following甩负荷load shedding, load rejection(控制棒等的)插入insertion(控制棒等的)抽出withdrawal反应堆调节系统Reactor Regulating System (RRS)(蒸汽发生器)排污、下泄blowdown 规程procedure技术规格technical specification瞬态,瞬变transient安装调试installation and commissioning冷态试验 cold functional test热态试验 hot functional test(反应堆)启动试验(reactor) start up test退役decommissioning主控室main control room方位角偏差azimuthal tilt径向功率分布radial power distribution轴向功率分布axial power distribution燃料管理方案fuel management scheme燃料-包壳交互作用fuel-clad interaction effect芯块-包壳交互作用(PCI) pellet-clad interaction(燃料)栅格、晶格lattice积水垢fouling去污cleansing(水的)净化purify (v), purification (n)1.7. 反应堆安全核安全nuclear safety安全功能 safety function衰变热decay heat余热residual heat空泡系数void coefficient法律law法规regulation / code联邦管理法规Code of Federal Regulations (CFR)法案(美)Act导则guidance, guide安全1/2/3级safety class 1/2/3可靠性reliability容限,裕量,边界margin堆芯热裕量core thermal margin 堵管裕量tube plugging margin运行安全裕量operating margin标准,准则criterion,复数形式: criteria概率安全分析Probabilistic Safety Analysis (PSA)概率风险分析Probabilistic Risk Analysis (PRA)确定论安全分析deterministic safety analysis初步安全分析报告Preliminary Safety Analysis Report (PSAR)最终安全分析报告Final Safety Analysis Report (FSAR)安全评价报告safety evaluation report事件incident事故accident后果consequence严重事故severe accident堆芯损毁core damage堆芯融化core meltdown全厂断电station blackout冷却剂丧失事故(失水事故)Loss-of-coolant Accident (LOCA)反应性引入事故Reactivity Insertion Accident (RIA)未能紧急停堆的预计瞬变Anticipated Transient Without Scram (ATWS)失电Loss of Power失流Loss of flow先漏后破leak before break (LBB)故障安全,失效保护fail-safe单一故障准则single-failure criterion共因故障common cause failure固有安全性inherent safety非能动安全passive safety冗余性redundancy多样性diversity多层屏障multiple barrier纵深防御defense in depth潜热latent heat 焓,热函sensible heat, enthalpy定期安全检查periodic safety inspection许可证license监管regulation核安全准则nuclear safety criteria三哩岛事故Three Mile Island (TMI) accident切尔诺贝利事故Chernobyl accident工作不正常,故障malfunction失效failure假设始发事件postulated initiating events 事故工况accident condition严重事故severe accident事故处理accident management设计基准事故design basis accident负荷丧失事故loss of electrical load accident主给水丧失事故loss of main feed water accident卡棒事故stuck rod accident弹棒事故rod ejection accident堵管裕量tube plugging margin管道甩摆限制pipe whip restraint在役检查inservice inspection承压热冲击pressurized thermal shock1.8. 放射性与辐射防护放射性radioactivity散射scatter折射deflect, deflection衍射diffraction穿透penetrate, penetration交互作用interact, interaction电离ionization湮灭annihilate (v.), annihilation (n.) 衰减attenuate(v.), attenuation(n.)入射离子projectile辐射防护radiation protection辐射,射线radiation, ray辐照,(向外)辐射irradiation 内照射internal exposure外照射external exposure职业照射(剂量)occupational dose 注量fluence宇宙射线cosmic rayX射线x-rayα/β/γ射线α/β/γray保健物理health physics屏蔽shielding生物屏蔽biological shield剂量dose剂量当量dose equivalent集体剂量collective dose个人剂量individual dose摄入,摄取ingest, ingestion 吸入inhale, inhalation昏迷coma绞痛cramp腹泻diarrhea颤抖tremor呕吐vomit身体的somatic躯体反应somatic effect辐照病变radiation sickness 征兆symptom治疗therapy 幸存者survivor活化产物activation product废水effluent天然本底natural background希弗sivert谱spectrum雷姆rem氡radon合理可行尽量低as low as reasonably achievable (ALARA)放射性废物处理radioactive waste disposal 高放废物high-level (radioactive) waste低放废物low-level (radioactive) waste1.9. 有关机构国际原子能机构International Atomic Energy Agency (IAEA)美国机械工程师学会American Society of Mechanical Engineers (ASME)美国核管会Nuclear Regulatory Commission (NRC/USNRC)美国能源部Department Of Energy (DOE)世界核电运营者协会World Association of Nuclear Operators (WANO)国际辐射防护委员会International Commission on Radiological Protection (ICRP) 中国国家原子能机构China Atomic Energy Authority (CAEA)国家环保总局State Environment Protection Administration of China1.10. 其它术语1.10.1.表示方向横向lateral纵向longitudinal径向radial垂直于perpendicular to 垂直/立式安装vertically mounted 环向circumferential轴向axial周边periphery1.10.2.材料术语腐蚀corrosion应力腐蚀开裂stress corrosion cracking 断裂rapture, fracture, break破口breach肿胀swelling气蚀cavitation点蚀/孔蚀pitting 缝隙腐蚀crevice corrosion冲蚀erosion流动加速腐蚀FAC flow accelerated corrosion耗蚀wastage凹陷,凹痕(SG tube) dent疲劳fatigue老化ageing降级degradation磨损wear微动磨损fretting wear蠕变creep应力stress应变strain蠕变强度creep strength抗拉强度tensile strength屈服强度yield strength断裂强度rapture strength断裂韧性fracture toughness零延性转变温度nil-ductility transition temperature基准性能baseline property热应力thermal stress辐照肿胀irradiation swelling焊接weld堆焊deposit welding, overlaying, build-up welding, surface welding密封焊seal weld对接焊butt weld焊缝weld seam焊接热影响区heat affected zone (HAZ)锻造forge锻件forging1.10.3.核电工程术语征购procurement安装erection调试commissioning厂址site招标call for bid, call for tender 商务标commercial offer技术标technical offer开工commencement合同contract不可更改的合同firm contract 分包合同subcontract承诺,任务commitment工地,工作现场job site 技术要求technical requirement技术规格technical specification不符合项non-conformance交货delivery仓库warehouse施工计划construction schedule离岸价格Free on Board (FOB)到岸价格Cost Insurance and Freight (CIF) EPC合同-设计采购建造,Engineering, Procurement & Construction电网power grid输变电系统distribution system1.10.4.其它化合物compound混合物mixture质量mass动量momentum能量energy势能potential (energy)动能kinetic energy惯性inertia半衰期half-life平均自由程mean free path 燃料循环fuel cycle 热点hot spot热管因子hot-channel factor偏离泡核沸腾比departure from nucleate boiling ratio (DNBR)传热heat transfer换热器heat exchanger导热heat conduction对流convection热辐射thermal / heat radiation干度quality蒸汽steam预应力钢筋混凝土prestressed reinforced concrete / prestressed concrete钢筋混凝土reinforced concrete铁钢沙混凝土Steel shot concrete筋,钢筋束tendon流量分配flow distribution电网power grid公共事业,业主utility业主owner承包商contractor分包商sub-contractor压降pressure drop压差differential pressure水位(water) level水位指示level indication 规定,条款;保障;装备provision地震earthquake地震的seismic飓风tornado暖通空调Heating, Ventilation and Air Conditioning (HV AC)热阱heat sink惰转coastdown惰转流量coastdown flow功率失常激增,功率漂移power excursion 减轻,缓解v. mitigate, n. mitigation公差、容差allowance间隙,公差clearance权重因子weighting factor1.11. 有必要了解的词汇与短语根据经验as a rule of thumb以数量级by orders of magnitude早期故障, 初期故障incipient failure消除eliminate, elimination导出,起源derive, derivation, deduce, deduction机理,原理mechanism, approach, principle, theory敏感的susceptible敏感性susceptibility有毒的toxic 急剧的acute遗传inherit遗传性的hereditary高度altitude假定,假设postulate, postulation 可渗透的, 有渗透性的permeable 不可渗透的impermeable脆性破裂brittle fracture脆化embrittlement韧性toughness延展性ductility1.12. AP1000核电厂相关术语非能动余热导出系统passive decay heat removal system爆破阀squib valve第2章阅读理解题练习2.1. Passage 1As a result reactor designers have paid great attention to the inherent safetyof reactors which can be achieved by negative temperature and power coefficients and fail-safe control systems. It can be said with some confidence that present-day thermal reactors are safe in the sense that under no conceivable circumstance can they explode like a bomb, and control systems have been designed which can, in the event of any malfunction on the part of the reactor or its associated plant, automatically and rapidly shut down the reactor, i.e. make it subcritical by a substantial amount, in a very few seconds.1. According to the paragraph, inherent safety of reactors can be achieved by . (C)A. the operators;B. positive temperature and positive power coefficients;C. negative temperature and negative power coefficients;D. passive safety system and positive power coefficients.2. The best title of the passage may probably be . (D)A. fail-safe control system;B. thermal reactor safety and operation;C. automatic protective system;D. inherent safety design of reactors.2.2. Passage IIThe biological shield should contain some hydrogen compound to slow down fast neutrons, and be dense enough to attenuate gamma radiation effectively. Concrete satisfies both these requirements fairly well and is suitable for landbase reactors. Barytes (重晶石) concrete, containing the heavy element barium, and steel-shot concrete have been used for biological shields. They are more dense than ordinary concrete, with improved shielding properties, however their higher cost offsets this advantage. The biological shield for a marine reactor, which is usually a fairly compact pressurized water reactor, must satisfy a minimum space and weight requirement. This leads to a shield design which consists typically of alternate layers of water (for fast neutron slowing) and steel (for gamma ray attenuation).3. According to the above passage, is not possible for constructing biological shield? (C)A. steel;B. concrete;C. graphite;D. paraffin wax.4. Which of the following sentences is not true? (D)A. The biological shield is designed mainly to slow down fast neutrons and attenuate gamma radiation.B. The marine reactor uses alternative steel and water layers as its biological shield.C. The combination of heavy element concrete and steel bars could improve the shielding properties.D. The biological shield should use hydrogen element to slow down fast neutron and attenuate gamma radiations.2.3. Passage IIIIn order to mitigate the effects of large release of steam (an potentially of radioactivity) in the containment, two full capacity independent safety systems are provided; the reactor building spray system and the reactor building emergency coolers. The systems are designed to provide cool water to condense discharge steam and to prevent containment pressure from reaching its design limit. Individual systems differ considerably but a typical system may be described as follows; The initial capacity of the systems in removing heat from the containment atmosphere is typically 253GJ/hr.When a containment pressure of 4psig is reached, the emergency coolers of the reactor building are actuated. In their post accident mode, the system consists of three units each with a fan and an emergency cooler. As the reactor building air is circulated across a tubular heat exchanger, a portion of steam is condensed. These coolers alone would be capable of returning the containment pressure to near atmospheric within 24 hr after an accident. When the pressure reaches a level of 10 psig, the second safety system, the reactor building spray system, is automatically actuated. It consists of a pump, piping, headers, and spray nozzles arranged uniformly under the containment dome. It can spray borated water into the reactor building at a rate of 11.35m3/min. A sodium hydroxide additive is also provided in the spray water to increase the retention of iodine, and hence, to reduce its concentration in the containment atmosphere in the event of a sizable breach of fuel cladding.5. Two full capacity independent safety systems are provided for the design purpose of . (A)A. condensing the steam released into the containment when pressureexceeds design limit.B. maintaining the high pressure in the containmentC. discharging large amount of steamD. mitigating the effect of radiation hazard to the containment.6. The reactor building spray system will be actuated automatically . (C)A. after 24 hours after the accident;B. immediately after the accident;C. when the containment pressure reaches a level of design limit;D. when the air in the containment is circulated through the heat exchanger.7. Which of the following statements is INCORRECT? (B)A. The emergency cooler consists of fans and heat exchangers.B. The emergency cooler system can spray borated water into containment.C. All the two system are applied for returning the containment pressure to be blow atmospheric after the accident.D. Sodium hydroxide additive is provided in the spray water to reduce the amount of radioactive fission produces.2.4. Passage IVMany reactor-years of operating experience have shown that it is not the fission chain reaction in the reactor core that is the most likely source of malfunction and accidents, but the “conventional”components of the power plant such as pumps, valves, switches, relays and parts under stress such as pressure vessel or pipework. Human error on the part of operating and maintenance staff has also proved to be a rather frequent source of trouble in nuclear power plant.These factors are not peculiar to nuclear power plant, but they assume great importance because of the hazardous nature of nuclear reactors. Designers have to ensure that all systems should as far as possible be fail-safe and redundant, i.e. if one system fails to function correctly, another is available to fulfill the same function.As stated above, nuclear reactors cannot explode like nuclear bombs. This primarily because of the fast acting negative thermal feedback due to Doppler broadening of the 238U absorption resonances. In addition in thermal reactors where neutrons are moderated, the prompt neutron lifetime Lp is the order of 10-4 second; in a bomb, since the neutrons are unmoderated, the prompt neuron lifetime is of the order of 10-8 seconds. Finally, reactor fuel consists typically of 2to 3 percent 235U, where as nuclear weapons contain almost pure 239Pu. The net effect of these difference is that, even in a reactor which is totally out of control and gone prompt critical, the reactor period will not be much less than a second or so. In a nuclear bomb, the period is of the order of nanoseconds.(1)According to the text, the most likely source of troubles in a nuclear comes fromA.Core of the reactorB.Conventional componentsC.Parts under stressD.Human error(2)The first sentence of second paragraph “These factors are not peculiar to …”,what does “These factors” mean?A.Human errorB.Malfunction of conventional componentsC.Fission chain reaction in the coreD.All of the above(3)What make the pressurized water reactors can not explode like a nuclear bomb?A.Doppler broadening of the 238U absorption resonancesB.The neutrons are moderatedC.PWR fuel contains much less fissile isotopes than nuclear bombD.All of the above(4)The last two sentences “The net effect of … is of the order of nanoseconds.” Givea comparison about the reactor period, what does the author most likely toexpress?A.The reactor period is too short comparing with a nuclear bombB.The 239Pu in a nuclear bomb is more easily to go prompt criticalityC.The nuclear reactor can not generate large amount of heat as a nuclear bombdoes within very short time, therefore nuclear reactor can not explode.D.The author wants to give us a reference about the data of the reactor periodtime.2.5. Passage VThe primary functions of the control rod drive mechanisms (CRDM) are to insert or withdraw rod cluster control assemblies and gray rod control assembles into or from the core to control average core temperature at a designed speed. A schematic diagram of CRDM is given in Fig 1. Control rod withdrawal one step involves six actions.1) Moveable Gripper Coil B-on.The latch-locking plunger rises andswings the movable gripper latchesinto the drive rod assembly groove. Asmall axial clearance exists betweenthe latch teeth and the drive rod.2) Stationary Gripper Coil A-off.The force of gravity, acting upon thedrive rod assembly and attachedcontrol rod, causes the stationarygripper and plunger to movedownward 1/16 inch, transferring theload of the drive rod assembly andattached control rod to the movablegripper latches. The plungerFig 1. Control rod drive mechanism continues to move downward andswings the stationary gripper latches out of the drive rod assembly groove.3) Lift Coil C-on. The 5/8-inch gap between the movable gripper pole and the lift pole closes, and the drive rod assembly rises one step length.4) Stationary Gripper Coil A-on. The plunger rises and rises the gap below the stationary gripper pole. The three links, pinned to the plunger, swing the stationary gripper latches into a drive rod assembly groove. The latches contact the drive rod assembly and lift it a small fraction of an inch. The small vertical drive rod assembly movement transfers the drive rod assembly load from the movable gripper latches to the stationary gripper latches.5) Movable Gripper Coil –off. The latch-locking plunger separates from the movable gripper pole under the force of a spring and gravity. Three links, pinned to the plunger, swing the three movable gripper latches out of the drive rod assembly groove.6) Lift Coil C –off. The gap between the movable gripper pole and the life pole opens. The movable gripper latches drop 5/8 inch to a position adjacent to a drive rod assemble groove.Repetition of the above six actions will make another step of withdrawal movement of control rod.(1)According to the text, the most likely source of troubles in a nuclear comes fromA.Core of the reactorB.Conventional componentsC.Parts under stressD.Human error(2)According to the text, the most likely source of troubles in a nuclear comes fromA.Core of the reactorB.Conventional componentsC.Parts under stressD.Human error第3章句子翻译-举例3.1. Which 从句(1)The atoms of all elements, which at one time were thought to be thefundamental particles of nature, consist of numbers of three more fundamental particles-protons, neutrons and electrons. 曾经被认为是自然界中基础粒子的原子是由多个更基础的粒子组成-质子、中子和电子。
羟胺与氰基反应
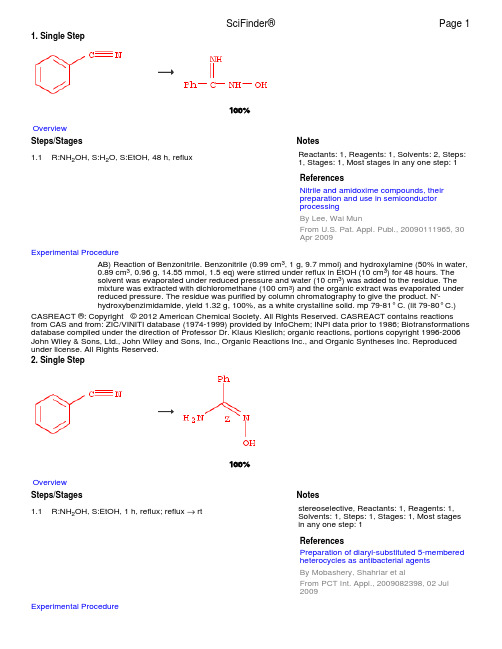
1. Single Step100%OverviewSteps/Stages Notes1.1 R:NH2OH, S:H2O, S:EtOH, 48 h, reflux Reactants: 1, Reagents: 1, Solvents: 2, Steps:1, Stages: 1, Most stages in any one step: 1ReferencesNitrile and amidoxime compounds, theirpreparation and use in semiconductorprocessingBy Lee, Wai MunFrom U.S. Pat. Appl. Publ., 20090111965, 30Apr 2009Experimental ProcedureAB) Reaction of Benzonitrile. Benzonitrile (0.99 cm3, 1 g, 9.7 mmol) and hydroxylamine (50% in water,0.89 cm3, 0.96 g, 14.55 mmol, 1.5 eq) were stirred under reflux in EtOH (10 cm3) for 48 hours. Thesolvent was evaporated under reduced pressure and water (10 cm3) was added to the residue. Themixture was extracted with dichloromethane (100 cm3) and the organic extract was evaporated underreduced pressure. The residue was purified by column chromatography to give the product. N'-hydroxybenzimidamide, yield 1.32 g, 100%, as a white crystalline solid. mp 79-81° C. (lit 79-80° C.) CASREACT ®: Copyright © 2012 American Chemical Society. All Rights Reserved. CASREACT contains reactions from CAS and from: ZIC/VINITI database (1974-1999) provided by InfoChem; INPI data prior to 1986; Biotransformations database compiled under the direction of Professor Dr. Klaus Kieslich; organic reactions, portions copyright 1996-2006 John Wiley & Sons, Ltd., John Wiley and Sons, Inc., Organic Reactions Inc., and Organic Syntheses Inc. Reproduced under license. All Rights Reserved.2. Single Step100%OverviewSteps/Stages Notes1.1 R:NH2OH, S:EtOH, 1 h, reflux; reflux → rt stereoselective, Reactants: 1, Reagents: 1,Solvents: 1, Steps: 1, Stages: 1, Most stagesin any one step: 1ReferencesPreparation of diaryl-substituted 5-memberedheterocycles as antibacterial agentsBy Mobashery, Shahriar et alFrom PCT Int. Appl., 2009082398, 02 Jul2009Experimental Procedure(Z)-N'-hydroxybenzamidine (compound 17-structure shown below): A solution of ethanol (5.0 mL),benzonitrile (203 mg, 1.97 mmol) and hydroxylamine (520 mg, 7.87 mmol) were refluxed for 1 hour.The reaction was then cooled to room temperature and concentrated in vacuo to give the a clear oilwhich was taken to the next step without further purification (268 mg, 100%). 1H NMR (500 MHz,CDCL3) δ(ppm): 4.92 (2H, bs), 7.38-7.44 (3H, m), 7.62-7.65 (2H, m). 13C NMR (125 MHz, CDCL3)δ(ppm): 126.1 (CH), 128.9 (CH), 130.2 (CH), 132.6, 152.8. MS (FAB+): 137 (MH+). HRMS forC7H8N2O (MH+): calculated: 137.0715; found 137.0718.CASREACT ®: Copyright © 2012 American Chemical Society. All Rights Reserved. CASREACT contains reactions from CAS and from: ZIC/VINITI database (1974-1999) provided by InfoChem; INPI data prior to 1986; Biotransformations database compiled under the direction of Professor Dr. Klaus Kieslich; organic reactions, portions copyright 1996-2006 John Wiley & Sons, Ltd., John Wiley and Sons, Inc., Organic Reactions Inc., and Organic Syntheses Inc. Reproduced under license. All Rights Reserved.3. Single Step100%OverviewSteps/Stages Notes1.1 R:NH2OH, S:EtOH, 1 h, reflux; reflux → rt stereoselective, Reactants: 1, Reagents: 1,Solvents: 1, Steps: 1, Stages: 1, Most stagesin any one step: 1ReferencesPreparation of oxadiazole derivatives asantibacterial agentsBy Mobashery, Shahriar et alFrom PCT Int. Appl., 2009041972, 02 Apr2009Experimental Procedure(Z)-N'-hydroxybenzamidine (compound 17 - structure shown below): A solution of ethanol (5.0 mL),benzonitrile (203 mg, 1.97 mmol) and hydroxylamine (520 mg, 7.87 mmol) were refluxed for 1 hour.The reaction was then cooled to room temperature and concentrated in vacuo to give the a clear oilwhich was taken to the next step without further purification (268 mg, 100%). 1H NMR (500 MHz,CDCL3) δ(ppm): 4.92 (2H, bs), 7.38-7.44 (3H, m), 7.62-7.65 (2H, m). 13C NMR (125 MHz, CDCL3)δ(ppm): 126.1 (CH), 128.9 (CH), 130.2 (CH), 132.6, 152.8. MS (FAB+): 137 (MH+). HRMS forC7H8N2O (MH+): calculated: 137.0715; found 137.0718.CASREACT ®: Copyright © 2012 American Chemical Society. All Rights Reserved. CASREACT contains reactions from CAS and from: ZIC/VINITI database (1974-1999) provided by InfoChem; INPI data prior to 1986; Biotransformations database compiled under the direction of Professor Dr. Klaus Kieslich; organic reactions, portions copyright 1996-2006 John Wiley & Sons, Ltd., John Wiley and Sons, Inc., Organic Reactions Inc., and Organic Syntheses Inc. Reproduced under license. All Rights Reserved.4. Single Step99%OverviewSteps/Stages Notes1.2 R:Disodium carbonate, S:H2OReferencesDiscovery and SAR exploration of N-aryl-N-(3-aryl-1,2,4-oxadiazol-5-yl)amines aspotential therapeutic agents for prostatecancerBy Krasavin, Mikhail et alFrom Chemistry Central Journal, 4, No pp.given; 2010CASREACT ®: Copyright © 2012 American Chemical Society. All Rights Reserved. CASREACT contains reactions from CAS and from: ZIC/VINITI database (1974-1999) provided by InfoChem; INPI data prior to 1986; Biotransformations database compiled under the direction of Professor Dr. Klaus Kieslich; organic reactions, portions copyright 1996-2006 John Wiley & Sons, Ltd., John Wiley and Sons, Inc., Organic Reactions Inc., and Organic Syntheses Inc. Reproduced under license. All Rights Reserved.5. Single Step95%OverviewSteps/Stages Notes1.1 R:H2NOH-HCl, R:NaOH, S:H2O, 1 h, 30°C, pH 10; 2 h, reflux Reactants: 1, Reagents: 2, Solvents: 1, Steps:1, Stages: 1, Most stages in any one step: 1ReferencesTwo synthetic methods of 3,4-bis(3-nitrophenyl)furoxanBy Yang, Jian-ming et alFrom Hanneng Cailiao, 17(5), 527-530; 2009 CASREACT ®: Copyright © 2012 American Chemical Society. All Rights Reserved. CASREACT contains reactions from CAS and from: ZIC/VINITI database (1974-1999) provided by InfoChem; INPI data prior to 1986; Biotransformations database compiled under the direction of Professor Dr. Klaus Kieslich; organic reactions, portions copyright 1996-2006 John Wiley & Sons, Ltd., John Wiley and Sons, Inc., Organic Reactions Inc., and Organic Syntheses Inc. Reproduced under license. All Rights Reserved.6. Single Step95%OverviewSteps/Stages NotesReferencesSynthesis of 3,4-bis(3',5'-dinitrophenyl-1'-yl)furoxanBy Huo, Huan et alFrom Hecheng Huaxue, 17(2), 208-210; 2009 CASREACT ®: Copyright © 2012 American Chemical Society. All Rights Reserved. CASREACT contains reactions from CAS and from: ZIC/VINITI database (1974-1999) provided by InfoChem; INPI data prior to 1986; Biotransformations database compiled under the direction of Professor Dr. Klaus Kieslich; organic reactions, portions copyright 1996-2006 John Wiley & Sons, Ltd., John Wiley and Sons, Inc., Organic Reactions Inc., and Organic Syntheses Inc. Reproduced under license. All Rights Reserved.7. Single Step93%OverviewSteps/Stages Notes1.1 R:NH2OH, S:H2O, S:MeOH, > 1 min, 50°C; 3 h, reflux Reactants: 1, Reagents: 1, Solvents: 2, Steps:1, Stages: 1, Most stages in any one step: 1ReferencesQuinazoline derivatives as adrenergicreceptor antagonists and their preparation,pharmaceutical compositions and use in thetreatment of diseasesBy Sarma, Pakala Kumara Savithru et alFrom Indian Pat. Appl., 2005DE01706, 31Aug 2007CASREACT ®: Copyright © 2012 American Chemical Society. All Rights Reserved. CASREACT contains reactions from CAS and from: ZIC/VINITI database (1974-1999) provided by InfoChem; INPI data prior to 1986; Biotransformations database compiled under the direction of Professor Dr. Klaus Kieslich; organic reactions, portions copyright 1996-2006 John Wiley & Sons, Ltd., John Wiley and Sons, Inc., Organic Reactions Inc., and Organic Syntheses Inc. Reproduced under license. All Rights Reserved.8. Single Step92%OverviewSteps/Stages Notes1.1 R:NH2OH, R:Et3N, S:EtOH, rt stereoselective, Reactants: 1, Reagents: 2,Solvents: 1, Steps: 1, Stages: 1, Most stagesin any one step: 1ReferencesPotent inhibitors of lipoprotein-associatedphospholipase A2: Benzaldehyde O-heterocycle-4-carbonyloximeBy Jeong, Hyung Jae et alFrom Bioorganic & Medicinal ChemistryLetters, 16(21), 5576-5579; 2006 CASREACT ®: Copyright © 2012 American Chemical Society. All Rights Reserved. CASREACT contains reactions from CAS and from: ZIC/VINITI database (1974-1999) provided by InfoChem; INPI data prior to 1986; Biotransformations database compiled under the direction of Professor Dr. Klaus Kieslich; organic reactions, portions copyright 1996-2006 John Wiley & Sons, Ltd., John Wiley and Sons, Inc., Organic Reactions Inc., and Organic Syntheses Inc. Reproduced under license. All Rights Reserved.9. Single Step89%OverviewSteps/Stages Notes1.1 R:EtN(Pr-i)2, R:H2NOH-HCl, S:EtOH, 18 h, 80°C Reactants: 1, Reagents: 2, Solvents: 1, Steps:1, Stages: 1, Most stages in any one step: 1ReferencesTuned methods for conjugate addition to avinyl oxadiazole; synthesis ofpharmaceutically important motifsBy Burns, Alan R. et alFrom Organic & Biomolecular Chemistry,8(12), 2777-2783; 2010CASREACT ®: Copyright © 2012 American Chemical Society. All Rights Reserved. CASREACT contains reactions from CAS and from: ZIC/VINITI database (1974-1999) provided by InfoChem; INPI data prior to 1986; Biotransformations database compiled under the direction of Professor Dr. Klaus Kieslich; organic reactions, portions copyright 1996-2006 John Wiley & Sons, Ltd., John Wiley and Sons, Inc., Organic Reactions Inc., and Organic Syntheses Inc. Reproduced under license. All Rights Reserved.10. Single Step91%OverviewSteps/Stages Notes1.1 R:NaOH, R:H2NOH-HCl, S:H2O, S:EtOH, 12 h, 80°C; cooled Reactants: 1, Reagents: 2, Solvents: 2, Steps:1, Stages: 1, Most stages in any one step: 1ReferencesPreparation of heteropolycyclic compoundsand their use as metabotropic glutamatereceptor antagonistsBy Edwards, Louise et alFrom U.S. Pat. Appl. Publ., 20050272779, 08Dec 2005Experimental ProcedureGeneral/Typical Procedure: Example 6 N-Hydroxy-3-methoxy-benzamidine. Using the generalprocedure of Shine et al., J. Heterocyclic Chem. (1989) 26:125-128, hydroxylamine hydrochloride (22ml, 5 M, 110 mmol) and sodium hydroxide (11 ml, 10 M, 110 mmol) were added to a solution of 3-methoxybenzonitrile (11.5 ml. 94 mmol) in ethanol (130 ml). The reaction mixture was then heated atreflux (80 °C.) for 12 h. After the mixture was cooled, most of the solvent was removed in vacuo. Thecrude product was partitioned between ethyl acetate and water, washed with saturated brine, driedover anhydrous sodium sulfate and the solvent was removed in vacuo. Flash chromatography on silicagel using 35-50% ethyl acetate in hexane yielded the title compound (8.05 g, 52%). Examples 7-9were prepared in an analogous method to the procedure given in Example 6. N-Hydroxy-benzamidine.N-hydroxy-benzamidine (4.83 g, 91%, white solid) was obtained from benzonitrile (4 g, 38.9 mmol),hydroxylamine hydrochloride (8.89 ml, 44.0 mmol) and sodium hydroxide (4.49 ml, 45.0 mmol) inethanol (30 ml). 1H NMR (CDCl3), δ (ppm): 8.81 (broad peak, 1H), 7.63 (m, 2H), 7.39(m, 3H), 4.91 (s,2H).CASREACT ®: Copyright © 2012 American Chemical Society. All Rights Reserved. CASREACT contains reactions from CAS and from: ZIC/VINITI database (1974-1999) provided by InfoChem; INPI data prior to 1986; Biotransformations database compiled under the direction of Professor Dr. Klaus Kieslich; organic reactions, portions copyright 1996-2006 John Wiley & Sons, Ltd., John Wiley and Sons, Inc., Organic Reactions Inc., and Organic Syntheses Inc. Reproduced under license. All Rights Reserved.11. Single Step91%OverviewSteps/Stages Notes1.1 R:NaOH, R:H2NOH-HCl, S:H2O, S:EtOH, 12 h, 80°C literature preparation, Reactants: 1, Reagents:2, Solvents: 2, Steps: 1, Stages: 1, Moststages in any one step: 1ReferencesPreparation of five-membered heterocycliccompounds as mGluR5 receptor antagonistsBy Wensbo, David et alFrom PCT Int. Appl., 2004014881, 19 Feb2004CASREACT ®: Copyright © 2012 American Chemical Society. All Rights Reserved. CASREACT contains reactions from CAS and from: ZIC/VINITI database (1974-1999) provided by InfoChem; INPI data prior to 1986; Biotransformations database compiled under the direction of Professor Dr. Klaus Kieslich; organic reactions, portions copyright 1996-2006 John Wiley & Sons, Ltd., John Wiley and Sons, Inc., Organic Reactions Inc., and Organic Syntheses Inc. Reproduced under license. All Rights Reserved.12. Single Step85%OverviewSteps/Stages Notes1.1 R:Et3N, R:H2NOH-HCl, S:EtOH, 18 h, reflux stereoselective (Z), Reactants: 1, Reagents: 2,Solvents: 1, Steps: 1, Stages: 1, Most stagesin any one step: 1ReferencesUnexpected C-C Bond Cleavage: Synthesisof 1,2,4-Oxadiazol-5-ones from Amidoximeswith Pentafluorophenyl or TrifluoromethylAnion Acting as Leaving GroupBy Gerfaud, Thibaud et alFrom Organic Letters, 13(23), 6172-6175;2011CASREACT ®: Copyright © 2012 American Chemical Society. All Rights Reserved. CASREACT contains reactions from CAS and from: ZIC/VINITI database (1974-1999) provided by InfoChem; INPI data prior to 1986; Biotransformations database compiled under the direction of Professor Dr. Klaus Kieslich; organic reactions, portions copyright 1996-2006 John Wiley & Sons, Ltd., John Wiley and Sons, Inc., Organic Reactions Inc., and Organic Syntheses Inc. Reproduced under license. All Rights Reserved.13. Single Step85%OverviewSteps/Stages Notes1.1 R:Disodium carbonate, R:H2NOH-HCl, S:H2O, S:EtOH, 15 min,55°Cultrasound (40kHz), reaction withoutultrasound at room temperature decreasedyield and increased reaction time, Reactants:1, Reagents: 2, Solvents: 2, Steps: 1, Stages:1, Most stages in any one step: 1ReferencesSynthesis of amidoximes using an efficientand rapid ultrasound methodBy Barros, Carlos Jonnatan Pimentel et alFrom Journal of the Chilean ChemicalSociety, 56(2), 721-722; 2011CASREACT ®: Copyright © 2012 American Chemical Society. All Rights Reserved. CASREACT contains reactions from CAS and from: ZIC/VINITI database (1974-1999) provided by InfoChem; INPI data prior to 1986; Biotransformations database compiled under the direction of Professor Dr. Klaus Kieslich; organic reactions, portions copyright 1996-2006 John Wiley & Sons, Ltd., John Wiley and Sons, Inc., Organic Reactions Inc., and Organic Syntheses Inc. Reproduced under license. All Rights Reserved.14. Single Step83%OverviewSteps/Stages Notes1.1 R:NaHCO3, R:H2NOH-HCl, S:H2O, S:EtOH, 4 h, 80°C Reactants: 1, Reagents: 2, Solvents: 2, Steps:1, Stages: 1, Most stages in any one step: 1ReferencesA novel bifunctional chelating agent based onbis(hydroxamamide) for 99mTc labeling ofpolypeptidesBy Ono, Masahiro et alFrom Journal of Labelled Compounds andRadiopharmaceuticals, 55(2), 71-79; 2012 CASREACT ®: Copyright © 2012 American Chemical Society. All Rights Reserved. CASREACT contains reactions from CAS and from: ZIC/VINITI database (1974-1999) provided by InfoChem; INPI data prior to 1986; Biotransformations database compiled under the direction of Professor Dr. Klaus Kieslich; organic reactions, portions copyright 1996-2006 John Wiley & Sons, Ltd., John Wiley and Sons, Inc., Organic Reactions Inc., and Organic Syntheses Inc. Reproduced under license. All Rights Reserved.15. Single Step80%OverviewSteps/Stages Notes1.1 R:NaHCO3, R:H2NOH-HCl, S:H2O, 10 min, 25°C1.2 S:EtOH, 20 h, 25°C1.3 R:H2NOH-HCl, 50 h, 25°Cregioselective, other product also detected, in-situ generated reagent, Reactants: 1,Reagents: 2, Solvents: 2, Steps: 1, Stages: 3,Most stages in any one step: 3ReferencesSynthesis, mechanism of formation, andmolecular orbital calculations ofarylamidoximesBy Srivastava, Rajendra M. et alFrom Monatshefte fuer Chemie, 140(11),1319-1324; 2009CASREACT ®: Copyright © 2012 American Chemical Society. All Rights Reserved. CASREACT contains reactions from CAS and from: ZIC/VINITI database (1974-1999) provided by InfoChem; INPI data prior to 1986; Biotransformations database compiled under the direction of Professor Dr. Klaus Kieslich; organic reactions, portions copyright 1996-2006 John Wiley & Sons, Ltd., John Wiley and Sons, Inc., Organic Reactions Inc., and Organic Syntheses Inc. Reproduced under license. All Rights Reserved.16. Single Step79%OverviewSteps/Stages Notes1.1 R:Disodium carbonate, R:H2NOH-HCl, S:H2O, S:EtOH Reactants: 1, Reagents: 2, Solvents: 2, Steps:1, Stages: 1, Most stages in any one step: 1ReferencesSynthesis of 1,2,4- and 1,3,4-oxadiazolesfrom 1-aryl-5-methyl-1H-1,2,3-triazole-4-carbonyl chloridesBy Obushak, N. D. et alFrom Russian Journal of Organic Chemistry,44(10), 1522-1527; 2008CASREACT ®: Copyright © 2012 American Chemical Society. All Rights Reserved. CASREACT contains reactions from CAS and from: ZIC/VINITI database (1974-1999) provided by InfoChem; INPI data prior to 1986; Biotransformations database compiled under the direction of Professor Dr. Klaus Kieslich; organic reactions, portions copyright 1996-2006 John Wiley & Sons, Ltd., John Wiley and Sons, Inc., Organic Reactions Inc., and Organic Syntheses Inc. Reproduced under license. All Rights Reserved.17. Single Step85%OverviewSteps/Stages Notes1.1 R:K2CO3, R:H2NOH-HCl, S:EtOH1.2 R:HCl, S:Et2O, S:H2O1.3 R:NH3, R:NaCl1.4 S:Et2OReactants: 1, Reagents: 5, Solvents: 3, Steps:1, Stages: 4, Most stages in any one step: 4ReferencesModification of the Tiemann rearrangement:One-pot synthesis of N,N-disubstitutedcyanamides from amidoximesBy Bakunov, Stanislav A. et alFrom Synthesis, (8), 1148-1159; 2000 CASREACT ®: Copyright © 2012 American Chemical Society. All Rights Reserved. CASREACT contains reactions from CAS and from: ZIC/VINITI database (1974-1999) provided by InfoChem; INPI data prior to 1986; Biotransformations database compiled under the direction of Professor Dr. Klaus Kieslich; organic reactions, portions copyright 1996-2006 John Wiley & Sons, Ltd., John Wiley and Sons, Inc., Organic Reactions Inc., and Organic Syntheses Inc. Reproduced under license. All Rights Reserved.18. Single Step76%OverviewSteps/Stages Notes1.1 R:EtN(Pr-i)2, R:H2NOH-HCl, S:EtOH, 6-12 h, 80°C Reactants: 1, Reagents: 2, Solvents: 1, Steps:1, Stages: 1, Most stages in any one step: 1ReferencesA versatile solid-phase synthesis of 3-aryl-1,2,4-oxadiazolones and analoguesBy Charton, Julie et alFrom Tetrahedron Letters, 48(8), 1479-1483;2007CASREACT ®: Copyright © 2012 American Chemical Society. All Rights Reserved. CASREACT contains reactions from CAS and from: ZIC/VINITI database (1974-1999) provided by InfoChem; INPI data prior to 1986; Biotransformations database compiled under the direction of Professor Dr. Klaus Kieslich; organic reactions, portions copyright 1996-2006 John Wiley & Sons, Ltd., John Wiley and Sons, Inc., Organic Reactions Inc., and Organic Syntheses Inc. Reproduced under license. All Rights Reserved.19. Single Step70%OverviewSteps/Stages Notes1.1 R:Disodium carbonate, R:H2NOH-HCl, S:H2O, S:EtOH, 8 h, reflux Reactants: 1, Reagents: 2, Solvents: 2, Steps:1, Stages: 1, Most stages in any one step: 1ReferencesDesign, synthesis, characterization, andantibacterial activity of {5-chloro-2-[(3-substitutedphenyl-1,2,4-oxadiazol-5-yl)-methoxy]-phenyl}-(phenyl)-methanonesBy Rai, Neithnadka Premsai et alFrom European Journal of MedicinalChemistry, 45(6), 2677-2682; 2010 CASREACT ®: Copyright © 2012 American Chemical Society. All Rights Reserved. CASREACT contains reactions from CAS and from: ZIC/VINITI database (1974-1999) provided by InfoChem; INPI data prior to 1986; Biotransformations database compiled under the direction of Professor Dr. Klaus Kieslich; organic reactions, portions copyright 1996-2006 John Wiley & Sons, Ltd., John Wiley and Sons, Inc., Organic Reactions Inc., and Organic Syntheses Inc. Reproduced under license. All Rights Reserved.20. Single Step70%OverviewSteps/Stages Notes1.1 R:H2NOH-HCl, R:NaHCO3, S:H2O, S:MeOH, 1 h, rt → 70°C; cooled stereoselective, Reactants: 1, Reagents: 2, Solvents: 2, Steps: 1, Stages: 1, Most stages in any one step: 1ReferencesDiscovery and Optimization of a Novel Series of N-Arylamide Oxadiazoles as Potent, Highly Selective and Orally Bioavailable Cannabinoid Receptor 2 (CB2) AgonistsBy Cheng, Yuan et alFrom Journal of Medicinal Chemistry, 51(16), 5019-5034; 2008Experimental ProcedureN-(9-Ethyl-9H-carbazol-3-yl)-3-(3-phenyl-1,2,4-oxadiazol-5-yl) propanamide (37). To a mixture ofsodium carbonate (1.0 g, 10 mmol) and hydroxylamine hydrochloride (1.0 g, 19 mmol) inmethanol/H2O was added benzonitrile (2 mL, 19 mmol). The mixture was heated to 70 °C for 1 h. Thecooled reaction mixture was concentrated, and the residue was taken up in dichloromethane. Theorganic layer was washed with water and concentrated to give (Z)-N'-hydroxybenzamidine (1.85 g,70% yield), which was used without further purification.CASREACT ®: Copyright © 2012 American Chemical Society. All Rights Reserved. CASREACT contains reactions from CAS and from: ZIC/VINITI database (1974-1999) provided by InfoChem; INPI data prior to 1986; Biotransformations database compiled under the direction of Professor Dr. Klaus Kieslich; organic reactions, portions copyright 1996-2006 John Wiley & Sons, Ltd., John Wiley and Sons, Inc., Organic Reactions Inc., and Organic Syntheses Inc. Reproduced under license. All Rights Reserved.21. Single Step75%OverviewSteps/Stages Notes1.1 R:H2NOH-HCl, R:Disodium carbonate, S:MeOH Reactants: 1, Reagents: 2, Solvents: 1, Steps:1, Stages: 1, Most stages in any one step: 1ReferencesN-Aryl N'-Hydroxyguanidines, A New Class ofNO-Donors after Selective Oxidation by NitricOxide Synthases: Structure-ActivityRelationshipBy Renodon-Corniere, Axelle et alFrom Journal of Medicinal Chemistry, 45(4),944-954; 2002Experimental ProcedureBenzamidoximes 30-32 were prepared by refluxing anhydrous methanolic solutions of hydroxylaminehydrochloride with the corresponding nitrile in the presence of sodium carbonate as previouslydescribed.57Benzamidoxime (30). Compound 30 was obtained as a white solid in 75% yield frombenzonitrile mp 76 °C (literature: 76 °C).57CASREACT ®: Copyright © 2012 American Chemical Society. All Rights Reserved. CASREACT contains reactions from CAS and from: ZIC/VINITI database (1974-1999) provided by InfoChem; INPI data prior to 1986; Biotransformations database compiled under the direction of Professor Dr. Klaus Kieslich; organic reactions, portions copyright 1996-2006 John Wiley & Sons, Ltd., John Wiley and Sons, Inc., Organic Reactions Inc., and Organic Syntheses Inc. Reproduced under license. All Rights Reserved.22. Single Step70%OverviewSteps/Stages Notes1.1 R:KOH, R:H2NOH-HCl, S:MeOH, 3-6 h, 6°C in-situ generated reagent, Reactants: 1,Reagents: 2, Solvents: 1, Steps: 1, Stages: 1,Most stages in any one step: 1ReferencesHCV NS5b RNA-Dependent RNAPolymerase Inhibitors: From α,γ-Diketoacidsto 4,5-Dihydroxypyrimidine- or 3-Methyl-5-hydroxypyrimidinonecarboxylic Acids. Designand SynthesisBy Summa, Vincenzo et alFrom Journal of Medicinal Chemistry, 47(22),5336-5339; 2004Experimental ProcedureN'-hydroxybenzenecarboximidamide (12), 3-(benzyloxy)-N'-hydroxybenzenecarboximidamide (13), N'-hydroxy-3-[(4-methoxybenzyl)oxy]benzenecarboximidamide were prepared from the correspondingnitriles by use of known procedures. Generally, one equiv of potassium hydroxide dissolved inmethanol was added to a solution of hydroxylamine hydrochloride (1 equiv) in methanol. Theprecipitated potassium chloride was removed by filtration and to the above solution the appropriate arylnitrile was added. Reaction mixture was stirred at 60°C for the appropriate time (3-6 h, TLCmonitoring). After cooling, the solvent was removed under vacuum, and the residue was triturated withdiethyl ether. The precipitate was collected and eventually recristallyzed from an appropriate solvent,furnishing the desired amidoxime in 60-70 % yield. N'-hydroxybenzenecarboximidamide (12): spectraldata matches literature data.3CASREACT ®: Copyright © 2012 American Chemical Society. All Rights Reserved. CASREACT contains reactions from CAS and from: ZIC/VINITI database (1974-1999) provided by InfoChem; INPI data prior to 1986; Biotransformations database compiled under the direction of Professor Dr. Klaus Kieslich; organic reactions, portions copyright 1996-2006 John Wiley & Sons, Ltd., John Wiley and Sons, Inc., Organic Reactions Inc., and Organic Syntheses Inc. Reproduced under license. All Rights Reserved.23. Single Step65%OverviewSteps/Stages Notes1.1 R:K2CO3, R:H2NOH-HCl, S:EtOH, 1 h, rt; 6 h, reflux Reactants: 1, Reagents: 2, Solvents: 1, Steps:1, Stages: 1, Most stages in any one step: 1ReferencesAcetic acid aldose reductase inhibitorsbearing a five-membered heterocyclic corewith potent topical activity in a visualimpairment rat modelBy La Motta, Concettina et alFrom Journal of Medicinal Chemistry, 51(11),3182-3193; 2008Experimental ProcedureGeneral Procedure for the Synthesis of N-Hydroxybenzimidamides3a-i and N-Hydroxy-2-phenylacetimidamides 4a-i. A solution of the appropriate nitrile 1a-i or 2a-i (1.00 mmol), hydroxylaminehydrochloride (1.35 mmol), and potassium carbonate (1.00 mmol) in ethanol was left under stirring atroom temperature for 1 h, then heated under reflux until the disappearance of the starting materials (6h, TLC analysis). After cooling, the resulting mixture was filtered and the solvent was evaporated todryness under reduced pressure to give the target compound as a white solid, which was purified byrecrystallization (Supporting Information, Tables 1 and 2).CASREACT ®: Copyright © 2012 American Chemical Society. All Rights Reserved. CASREACT contains reactions from CAS and from: ZIC/VINITI database (1974-1999) provided by InfoChem; INPI data prior to 1986; Biotransformations database compiled under the direction of Professor Dr. Klaus Kieslich; organic reactions, portions copyright 1996-2006 John Wiley & Sons, Ltd., John Wiley and Sons, Inc., Organic Reactions Inc., and Organic Syntheses Inc. Reproduced under license. All Rights Reserved.24. Single Step60%OverviewSteps/Stages Notes1.1 R:Et3N, R:H2NOH-HCl, S:EtOH, rt → reflux; 24 h, reflux Reactants: 1, Reagents: 2, Solvents: 1, Steps:1, Stages: 1, Most stages in any one step: 1ReferencesSynthesis and cannabinoid activity of 1-substituted-indole-3-oxadiazole derivatives:Novel agonists for the CB1 receptorBy Moloney, Gerard P. et alFrom European Journal of MedicinalChemistry, 43(3), 513-539; 2008 CASREACT ®: Copyright © 2012 American Chemical Society. All Rights Reserved. CASREACT contains reactions from CAS and from: ZIC/VINITI database (1974-1999) provided by InfoChem; INPI data prior to 1986; Biotransformations database compiled under the direction of Professor Dr. Klaus Kieslich; organic reactions, portions copyright 1996-2006 John Wiley & Sons, Ltd., John Wiley and Sons, Inc., Organic Reactions Inc., and Organic Syntheses Inc. Reproduced under license. All Rights Reserved.25. Single Step60%Overview。
制药工程专业英语考试题目及答案
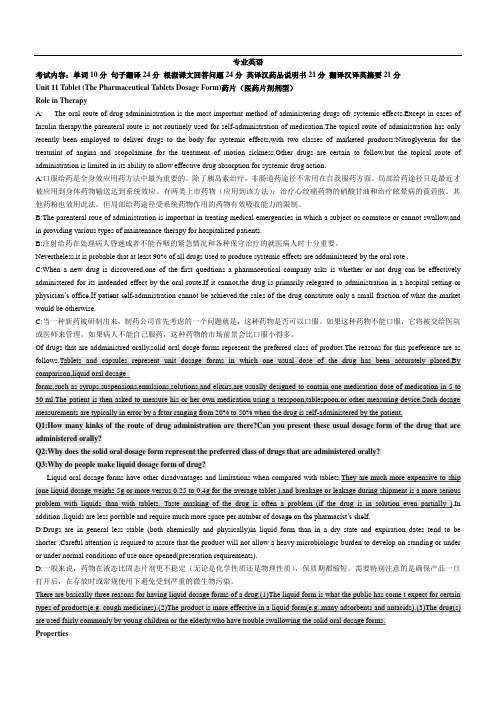
专业英语考试内容:单词10分句子翻译24分根据课文回答问题24分英译汉药品说明书21分翻译汉译英摘要21分Unit 11 Tablet (The Pharmaceutical Tablets Dosage Form)药片(医药片剂剂型)Role in TherapyA: The oral route of drug admininistration is the most important method of administering drugs ofr systemic effects.Except in cases of Insulin therapy.the parenteral route is not routinely used for self-administration of medication.The topical route of administration has only recently been employed to deliver drugs to the body for systemic effects,with two classes of marketed products:Nitroglycerin for the treatmint of angina and scopolamine for the treatment of motion sickness.Other drugs are certain to follow,but the topical route of administration is limited in its ability to allow effective drug absorption for systemic drug action.A:口服给药是全身效应用药方法中最为重要的。
除了胰岛素治疗,非肠道药途径不常用在自我服药方面。
核科学技术术语

核科学技术术语---裂变反应堆核科学技术术语---裂变反应堆(Glossary ofterms:nuclear science and technology-Fission reactor),并做了必要的修正。
它涉及了裂变反应堆领域有关的术语及定义。
反应堆堆型名词术语1.1 (核)反应堆(nuclear) reactor 能维持可控自持链式核裂变反应的装置。
注释:更广泛的意义上讲,反应堆这一术语应覆盖裂变堆、聚变堆、裂变聚变混合堆,但一般情况下仅指裂变堆。
1.2 动力(反应)堆power reactor 用于发电、推进和供热等用途的反应堆。
1.3 供热(反应)堆heating reactor 用于向居民和(或)工业设施等供热的反应堆。
1.4 研究(反应)堆research reactor 主要作基础研究或应用研究用的反应堆,例如:a. 高通量反应堆b. 脉冲反应堆c. 材料试验反应堆d. 零功率反应堆1.5 生产(反应)堆production reactor 主要用于生产易裂变材料的反应堆。
除另有说明外,通常指生产钚的反应堆。
1.6 增殖(反应)堆breeder reactor 转换比大于1的反应堆。
1.7 空间反应堆space reactor 将核裂变反应产生的能量转换成电能作为航天飞行器电源的一种核反应堆。
1.8 微型中子源反应堆miniature neutron source reactor 用高浓金属铀作燃料元件,金属铍作反射层,轻水慢化,自然对流冷却的一种作中子源用袖珍式核反应堆,可用于中子活化分析及少量研究用短寿命示踪同位素的制备。
1.9 零功率(反应)堆临界装置zero-power reactor;zero-energy reactor critical assembly 设计在极低功率下运行,不需要专门设置冷却剂系统的反应堆。
1.10 脉冲(反应)堆pulsed reactor 用于产生短持续时间、强中子脉冲的反应堆。
作文范文之新型能源英语作文
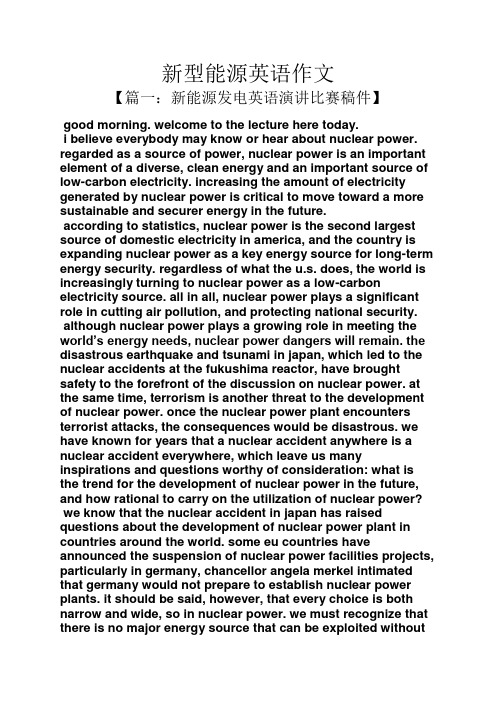
新型能源英语作文【篇一:新能源发电英语演讲比赛稿件】good morning. welcome to the lecture here today.i believe everybody may know or hear about nuclear power. regarded as a source of power, nuclear power is an important element of a diverse, clean energy and an important source of low-carbon electricity. increasing the amount of electricity generated by nuclear power is critical to move toward a more sustainable and securer energy in the future.according to statistics, nuclear power is the second largest source of domestic electricity in america, and the country is expanding nuclear power as a key energy source for long-term energy security. regardless of what the u.s. does, the world is increasingly turning to nuclear power as a low-carbon electricity source. all in all, nuclear power plays a significant role in cutting air pollution, and protecting national security.although nuclear power plays a growing role in meeting the world’s energy needs, nuclear power dangers will remain. the disastrous earthquake and tsunami in japan, which led to the nuclear accidents at the fukushima reactor, have brought safety to the forefront of the discussion on nuclear power. at the same time, terrorism is another threat to the development of nuclear power. once the nuclear power plant encounters terrorist attacks, the consequences would be disastrous. we have known for years that a nuclear accident anywhere is a nuclear accident everywhere, which leave us many inspirations and questions worthy of consideration: what is the trend for the development of nuclear power in the future, and how rational to carry on the utilization of nuclear power?we know that the nuclear accident in japan has raised questions about the development of nuclear power plant in countries around the world. some eu countries have announced the suspension of nuclear power facilities projects, particularly in germany, chancellor angela merkel intimated that germany would not prepare to establish nuclear power plants. it should be said, however, that every choice is both narrow and wide, so in nuclear power. we must recognize that there is no major energy source that can be exploited withoutrisk. as long as we prohibit the abuse of nuclear power, and improve security to make use of its favorable factors, nuclear power will take more active part in improving living conditions and the ecological environment rather than destroying our home.in my view, given the worlds fast-growing energy needs, nuclear power should be properly developed due to its specialty in energy shortage supplement and environmental pollution reduction. under the safe and security premise, site selection of nuclear power plant should be places away from the population centre and where there are few or no natural geological hazards, such as earthquakes. in the transition of energy utilization, nuclear power can assume a major role to supply the power generation, and its development can be constrained after the technology maturity of wind power, solar power and other new energy power generation.while working to encourage the peaceful use of nuclear power, we must seek the security of a world without nuclear weapons and a peaceful expansion of nuclear power. working cooperatively and with a shared sense of commitment and obligation, we can succeed in building a clean and safer energy future, and without this support, nuclear power will not be able to play the right kind of role which it can and should play in the global energy future. i look forward to working with all of you gathered here today to turn this vision into a reality, and ultimately leave our children with a stronger, bettercountry than when we found it.thank you and i’m happy to take some questions.【篇二:机械英语作文】mechanical engineeringmechanical engineering is the science of natural science and technology as the theoretical basis of technical production practice experience, research and address the development, design, manufacture, installation, use and repair of all machinery in the application of theoretical and practical issues disciplines. machinery of modern society, the five elements of production and services (people, capital, energy, materials and machinery) of, and participation in energy and materials production. any modern industrial and mechanical engineeringapplications are required, such as agriculture, forestry, mining and other necessary agricultural machinery, forestry machinery, mining equipment; metallurgy and chemical industry needs metallurgical machinery, chemical machinery; textiles and food processing industry requires, textile machinery, food processing machinery; housing construction and roads, bridges, water and other projects required construction machinery; power industry need to power machinery; transportation needs of various types of vehicles, ships, aircraft, etc.; the measurement of a variety of goods, packaging, storage and handling needs of the corresponding working machinery. is the peoples daily lives, more and more application of machinery, such as the crusher, cars, bicycles, sewing machines, watches, cameras, washing machines, refrigerators, air conditioners, vacuum cleaners, and so on.the development of various engineering mechanical engineering are required to have a corresponding development of mechanical engineering are required to provide the necessary machinery. some mechanical invention and improvement has led to new engineering technologies and the emergence of new industries and development, such as large power machinery manufacturing success, led to the establishment of the power system; locomotive and railway invention led to the rise of railway undertakings ; internal combustion engines, gas turbines, rocket engines of invention and progress as well as aircraft and spacecraft led to the successful development of aviation, aerospace engineering and aviation, the rise of the aerospace industry; high-pressure equipment (including compressors, reactors, sealing technology, etc.) development synthetic chemistry has led to many new projects success. mechanical engineering is increasing in all areas under the pressure of demand to gain traction, same time and from various disciplines and technological advances in improved and innovative capacity.as a school of mechanical engineering in college, i consider mechanical and automation is a very difficult profession, in our school curriculum is a lot of people complain about more difficult, but to learn the mechanical and automation on the premise that those specialized good understand, and then after a lot of practice learning opportunities, grasp, morehands, more brains, not to indulge in those old knowledge to learn to think more about your mind to think about thosethings usually do not ask the teacher to know more, four in the big three to take to participate in the internship, what the teacher does not know how to communicate as soon as possible and do not pressure in the heart, drawing, the physical, computer must learn learn these fine, hope that wecan learn this door is very difficult course, do not give away because of pressure of course!翻译:机械工程机械工程是以有关的自然科学和技术科学为理论基础,结合生产实践中的技术经验,研究和解决在开发、设计、制造、安装、运用和修理各种机械中的全部理论和实际问题的应用学科。
翻译(排序版)核专业英语~nuclear energy
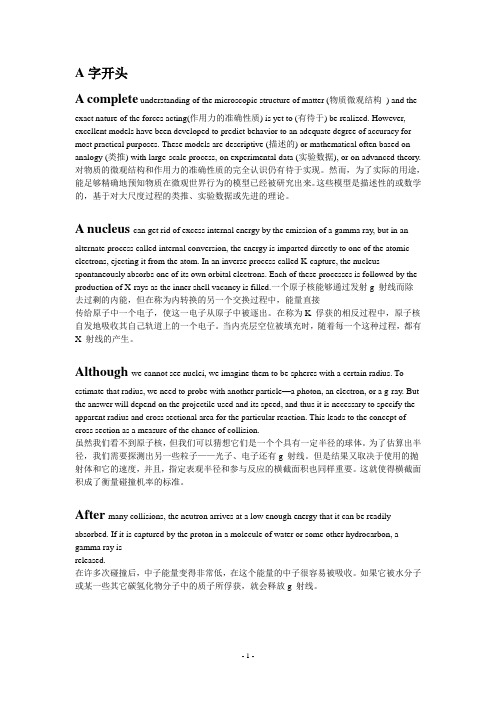
A字开头A complete understanding of the microscopic structure of matter (物质微观结构) and the exact nature of the forces acting(作用力的准确性质) is yet to (有待于) be realized. However, excellent models have been developed to predict behavior to an adequate degree of accuracy for most practical purposes. These models are descriptive (描述的) or mathematical often based on analogy (类推) with large-scale process, on experimental data (实验数据), or on advanced theory.对物质的微观结构和作用力的准确性质的完全认识仍有待于实现。
然而,为了实际的用途,能足够精确地预知物质在微观世界行为的模型已经被研究出来。
这些模型是描述性的或数学的,基于对大尺度过程的类推、实验数据或先进的理论。
A nucleus can get rid of excess internal energy by the emission of a gamma ray, but in analternate process called internal conversion, the energy is imparted directly to one of the atomic electrons, ejecting it from the atom. In an inverse process called K-capture, the nucleus spontaneously absorbs one of its own orbital electrons. Each of these processes is followed by the production of X-rays as the inner shell vacancy is filled.一个原子核能够通过发射g 射线而除去过剩的内能,但在称为内转换的另一个交换过程中,能量直接传给原子中一个电子,使这一电子从原子中被逐出。
化学化工专业英语翻译 reactor types
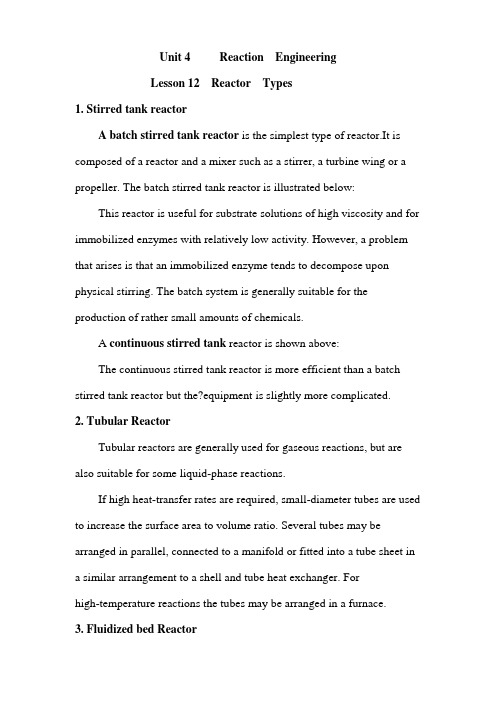
Unit 4 Reaction EngineeringLesson 12 Reactor Types1. Stirred tank reactorA batch stirred tank reactor is the simplest type of reactor.It is composed of a reactor and a mixer such as a stirrer, a turbine wing or a propeller. The batch stirred tank reactor is illustrated below:This reactor is useful for substrate solutions of high viscosity and for immobilized enzymes with relatively low activity. However, a problem that arises is that an immobilized enzyme tends to decompose upon physical stirring. The batch system is generally suitable for the production of rather small amounts of chemicals.A continuous stirred tank reactor is shown above:The continuous stirred tank reactor is more efficient than a batch stirred tank reactor but the?equipment is slightly more complicated.2. Tubular ReactorTubular reactors are generally used for gaseous reactions, but are also suitable for some liquid-phase reactions.If high heat-transfer rates are required, small-diameter tubes are used to increase the surface area to volume ratio. Several tubes may be arranged in parallel, connected to a manifold or fitted into a tube sheet in a similar arrangement to a shell and tube heat exchanger. Forhigh-temperature reactions the tubes may be arranged in a furnace.3. Fluidized bed ReactorA fluidized bed reactor (FBR) is a type of reactor device that can be used to carry out a variety of multiphase chemical reactions. In this type of reactor, a fluid (gas or liquid) is passed through a granular solid material (usually a catalyst possibly shaped as tiny spheres) at high enough velocities to suspend the solid and cause it to behave as though it were a fluid. This process, known as fluidization, imparts many important advantages to the FBR. As a result, the fluidized bed reactor is now used in many industrial applications.(1)Basic principlesThe solid substrate (the catalytic material upon which chemical species react) material in the fluidized bed reactor is typically supported by a porous plate, known as a distributor. The fluid is then forced through the distributor up through the solid material. At lower fluid velocities, the solids remain in place as the fluid passes through the voids in the material. This is known as a packed bed reactor. As the fluid velocity is increased, the reactor will reach a stage where the force of the fluid on the solids is enough to balance the weight of the solid material. This stage is known as incipient fluidization and occurs at this minimum fluidization velocity. Once this minimum velocity is surpassed, the contents of the reactor bed begin to expand and swirl around much like an agitated tank or boiling pot of water. The reactor is now a fluidized bed. Depending on the operating conditions and properties of solid phase various flow regimes can be observed in this reactor.(2)AdvantagesThe increase in fluidized bed reactor use in today’s industrial world is largely due to the inherent advantages of the technology.●Uniform Particle Mixing:Due to the intrinsic fluid-like behavior of the solid material, fluidized beds do not experience poor mixing as in packed beds. This complete mixing allows for a uniform product that can often be hard to achieve in other reactor designs. The elimination of radial and axial concentration gradients also allows for better fluid-solid contact, which is essential for reaction efficiency and quality.●Uniform Temperature Gradients:Many chemical reactions produce or require the addition of heat. Local hot or cold spots within the reaction bed, often a problem in packed beds, are avoided in a fluidized situation such as an FBR. In other reactor types, these local temperature differences, especially hotspots, can result in product degradation. Thus FBRs are well suited to exothermic reactions. Researchers have also learned that the bed-to-surface heat transfer coefficients for FBRs are high.●Ability to Operate Reactor in Continuous State:The fluidized bed nature of these reactors allows for the ability to continuously withdraw product and introduce new reactants into the reaction vessel. Operating at a continuous process state allows manufacturers to produce their various products more efficiently due tothe removal of startup conditions in batch processes.( 3 ) DisadvantagesAs in any design, the fluidized bed reactor does have it draw-backs, which any reactor designer must take into consideration.●Increased Reactor Vessel Size:Because of the expansion of the bed materials in the reactor, a larger vessel is often required than that for a packed bed reactor. This larger vessel means that more must be spent on initial startup costs.●Pumping Requirements and Pressure Drop:The requirement for the fluid to suspend the solid material necessitates that a higher fluid velocity is attained in the reactor. In order to achieve this, more pumping power and thus higher energy costs are needed. In addition, the pressure drop associated with deep beds also requires additional pumping power.●Particle Entrainment:The high gas velocities present in this style of reactor often result in fine particles becoming entrained in the fluid. These captured particles are then carried out of the reactor with the fluid, where they must be separated. This can be a very difficult and expensive problem to address depending on the design and function of the reactor. This may often continue to be a problem even with other entrainment reducing technologies.●Lack of Current Understanding:Current understanding of the actual behavior of the materials in a fluidized bed is rather limited. Itis very difficult to predict and calculate the complex mass and heat flows within the bed. Due to this lack of understanding, a pilot plant for new processes is required. Even with pilot plants, the scale-up can be very difficult and may not reflect what was experienced in the pilot trial.●Erosion of Internal Components: The fluid-like behavior of the fine solid particles within the bed eventually results in the wear of the reactor vessel. This can require expensive maintenance and upkeep for the reaction vessel and pipes.4. Packed bed ReactorThere are two basic types of packed-bed reactor: those in which the solid is a reactant, and those in which the solid is a catalyst. Many examples of the first type can be found in the extractive metallurgical industries.In the chemical process industries the designer will normally be concerned with the second type: catalytic reactors. Industrial packed-bed catalytic reactors range in size from small tubes, a few centimeters diameter to large diameter packed beds. Packed-bed reactors are used for gas and gas-liquid reactions. Heat-transfer rates in large diameter packed beds are poor and where high heat-transfer rates are required fluidized beds should be considered.Unit 4 REACTION ENGINEERINGLESSON 12 REACTOR TYPES1.搅拌反应釜间歇搅拌反应釜是最简单的反应釜类型。
Materials challenges in nuclear energy
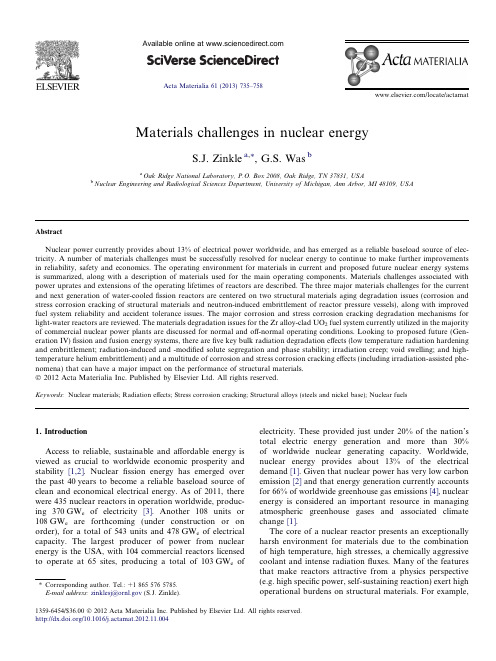
Materials challenges in nuclear energyS.J.Zinkle a ,⇑,G.S.Was baOak Ridge National Laboratory,P.O.Box 2008,Oak Ridge,TN 37831,USAbNuclear Engineering and Radiological Sciences Department,University of Michigan,Ann Arbor,MI 48109,USAAbstractNuclear power currently provides about 13%of electrical power worldwide,and has emerged as a reliable baseload source of elec-tricity.A number of materials challenges must be successfully resolved for nuclear energy to continue to make further improvements in reliability,safety and economics.The operating environment for materials in current and proposed future nuclear energy systems is summarized,along with a description of materials used for the main operating components.Materials challenges associated with power uprates and extensions of the operating lifetimes of reactors are described.The three major materials challenges for the current and next generation of water-cooled fission reactors are centered on two structural materials aging degradation issues (corrosion and stress corrosion cracking of structural materials and neutron-induced embrittlement of reactor pressure vessels),along with improved fuel system reliability and accident tolerance issues.The major corrosion and stress corrosion cracking degradation mechanisms for light-water reactors are reviewed.The materials degradation issues for the Zr alloy-clad UO 2fuel system currently utilized in the majority of commercial nuclear power plants are discussed for normal and off-normal operating conditions.Looking to proposed future (Gen-eration IV)fission and fusion energy systems,there are five key bulk radiation degradation effects (low temperature radiation hardening and embrittlement;radiation-induced and -modified solute segregation and phase stability;irradiation creep;void swelling;and high-temperature helium embrittlement)and a multitude of corrosion and stress corrosion cracking effects (including irradiation-assisted phe-nomena)that can have a major impact on the performance of structural materials.Ó2012Acta Materialia Inc.Published by Elsevier Ltd.All rights reserved.Keywords:Nuclear materials;Radiation effects;Stress corrosion cracking;Structural alloys (steels and nickel base);Nuclear fuels1.IntroductionAccess to reliable,sustainable and affordable energy is viewed as crucial to worldwide economic prosperity and stability [1,2].Nuclear fission energy has emerged over the past 40years to become a reliable baseload source of clean and economical electrical energy.As of 2011,there were 435nuclear reactors in operation worldwide,produc-ing 370GW e of electricity [3].Another 108units or 108GW e are forthcoming (under construction or on order),for a total of 543units and 478GW e of electrical capacity.The largest producer of power from nuclear energy is the USA,with 104commercial reactors licensed to operate at 65sites,producing a total of 103GW e ofelectricity.These provided just under 20%of the nation’s total electric energy generation and more than 30%of worldwide nuclear generating capacity.Worldwide,nuclear energy provides about 13%of the electrical demand [1].Given that nuclear power has very low carbon emission [2]and that energy generation currently accounts for 66%of worldwide greenhouse gas emissions [4],nuclear energy is considered an important resource in managing atmospheric greenhouse gases and associated climate change [1].The core of a nuclear reactor presents an exceptionally harsh environment for materials due to the combination of high temperature,high stresses,a chemically aggressive coolant and intense radiation fluxes.Many of the features that make reactors attractive from a physics perspective (e.g.high specific power,self-sustaining reaction)exert high operational burdens on structural materials.For example,1359-6454/$36.00Ó2012Acta Materialia Inc.Published by Elsevier Ltd.All rights reserved./10.1016/j.actamat.2012.11.004⇑Corresponding author.Tel.:+18655765785.E-mail address:zinklesj@ (S.J.Zinkle)./locate/actamatAvailable online atActa Materialia 61(2013)735–758the recoverable energy from each235Ufission reaction is $200MeV,which is about eight orders of magnitude per atom higher than typical chemical reactions.As a result, typical power densities in commercial nuclear reactor cores are$50–75MW th mÀ3,which is nearly two orders of mag-nitude higher than the average power density in the boiler furnace of a large-scale coal power plant.This intense pro-duction of heat is accompanied by the generation of ener-getic neutrons(which serve to sustain thefission reaction) and gamma radiation,which can degrade materials by dis-placement damage and radiolysis processes,respectively. Recent activities to extend the operating lifetime of current water reactors,to develop advancedfission reactor con-cepts with greater functionality and capability,and the coming emergence of fusion energy represent even greater demands on materials[5–8].1.1.Types of nuclearfission reactorsThe predominant reactor design worldwide is the pres-surized water reactor(PWR),accounting for two-thirds of the installed capacity,followed by boiling water reactors (BWRs)at21%and heavy-water reactors at14%of installed capacity,respectively(Table1)[3].All of these water-cooled reactors use ceramic fuel pellets consisting of UO2or otherfissile actinide oxides to generate heat. The ceramic pellets are stacked inside of long Zr alloy tubes (fuel cladding)that transfer the nuclear heat toflowing water coolant and serve as the primary barrier containing the volatile radioactivefission byproducts.The remaining 5%of installed nuclear energy comes from gas-cooled reac-tors,graphite-moderated reactors and liquid metal cooled reactors(Table1).The vast majority of the reactors listed in Table1are classified as Generation II reactors[9],which were designed in the1960s and predominantly achieved initial commercial operation from the1970s through the1990s.These reactors are distinguished from Generation I designs(1950s-60s), which were early commercial prototype and demonstration reactors,and Generation III reactors,designed in the1990s to incorporate significant advances in safety and economics [9].Generation III reactor construction for the past decade has been centered in Asia,with a few units recently built in Europe.The current generation of light-water reactors (LWRs),Generation III+,include still further advance-ment in economics and safety,such as passive heat removal systems.There are a total of108Generation III and Generation III+reactors on order or under construction around the world,and of those,89are PWRs.Given the high representation of PWRs and BWRs in the world’sfleet,materials issues in these two types of reac-tors are of greatest interest.And of the many materials in a reactor,those that experience the most extreme conditions (stress,corrosion,and radiation)are most important for maintaining plant safety and reliability.Fig.1shows a schematic of the major components in the primary and secondary circuits of a PWR[10].Pressurized water ($15.5MPa)in the primary circuit enters the reactor core at$275°C,picks up heat from the reactor core with a core exit temperature of$325°C,and transfers the heat across the U-tubes in the steam generator to water at a lower pressure.This water turns to steam that powers the tur-bine,and is condensed and recirculated.Fig.1also lists the alloys used throughout the primary and secondary cir-cuits,all of which are in contact with high-temperature water and are subject to significant mechanical stress. Alloys inside(and including)the reactor vessel are also subject to varying levels of radiation,which produces displacement damage and radiolytic decomposition of the coolant water.Major pressure boundary components (reactor pressure vessel,pressurizer,steam generator, steam lines,turbine and condenser)are made of either low carbon or low alloy steel.Austenitic stainless steels (Types304,304L,316,316L,321,347)dominate the core structural materials,as well as serving for cladding(308SS and309SS)on the inside surface of the reactor pressure vessel and pressurizer.Higher strength components such as springs and fasteners are made of nickel-base alloys. Vessel penetrations and steam generator tubes are made of nickel-base alloy690(previously alloy600,which was found to provide insufficient resistance to stress corrosion cracking).Condenser tubes are generally made of titanium or stainless steel.The selection of nickel-base alloys and austenitic stainless steels for core internals and the steam generator tubes is driven by the need for good aqueous corrosion resistance at high temperatures.These alloys have low corrosion rates due to the formation of chro-mium-bearing spinels that form adherent,high-density protective surface layers that grow very slowly at operating temperatures.Table1Power reactors by type,worldwide[3].Reactor type#Units Net MW e#Units Net MW e#Units Net MW e(in operation)(forthcoming)(total)Pressurized light-water reactors(PWR)267246555.18993,014356339569.1 Boiling light-water reactors(BWR)8478320.6680569086376.6 Gas-cooled reactors,all models178732.01200188932.0 Heavy-water reactors,all models5125610.0851125930722.0 Graphite-moderated reactors,all models1510219.0001510219.0 Liquid-metal-cooled reactors,all models1560.0410*******.0 Totals435369996.7108107,896543477894.7 736S.J.Zinkle,G.S.Was/Acta Materialia61(2013)735–758The main difference between PWRs and BWRs is that the latter consists of a single water circuit designed for boil-ing to occur in the core with steamflowing directly to the turbine,which eliminates the steam generator and pressur-izer found in the PWR.The operating temperatures are comparable for both reactor types($300°C),with compa-rable stress and radiation environments.As such,most of the structural alloys are very similar between the two reac-tor types.The main difference is in the zirconium alloys used as fuel rod cladding,with BWR fuel cladding opti-mized for corrosion resistance in higher oxygen potentials and PWR fuel cladding optimized for resistance to hydro-gen absorption in the low potential environment of the core.Typical zirconium alloy cladding materials used in BWR and PWR reactors are summarized in Table2. Differences in oxygen potential result in significant impacts on the stress corrosion degradation of materials through-out the water circuit in both reactor types,as will be discussed in Section2.1.The last reactor design that is in significant use world-wide is the pressurized heavy water reactor(PHWR),the most prevalent version being the CANDU(CANadian Deuterium Uranium)reactor.This reactor uses heavy water as the moderator and primary coolant,transferring heat to light water via a steam generator.The key charac-teristic of this reactor is the use of deuterium as a modera-tor,for which neutron absorption is low enough to permit the use of natural(unenriched)uranium,thus bypassing the need for expensive enrichment facilities.A major differ-ence in materials in this system vs.LWRs is the use of Zr–Nb pressure tubes that house the Zircaloy-clad fuel and the high pressure D2O.These tubesfit into Zircaloy-4calan-dria tubes that pass through a thin walled stainless steel calandria vessel,which also contains the lowtemperature Table2Summary of typical commercial zirconium alloys used as cladding in PWRs and BWRs.Reactor type Zr alloy composition Thermomechanical treatmentBWR Zircaloy-2(1.5%Sn–0.15%Fe–0.1%Cr–0.05%Ni)RecrystallizedPWR Zircaloy-4(1.5%Sn–0.2%Fe–0.1%Cr)Cold-worked and stress relief anneal PWR ZIRLO(1–2%Nb–1%Sn–0.1%Fe)Quench and temper/stress relief anneal PWR M5(1%Nb)RecrystallizedD2O moderator.Thus,zirconium alloys play a larger role as pressure boundary materials in PHWRs than they do in LWRs.Most reactors in the USA and elsewhere in the world were completed in the1970s and1980s,and today the aver-age age of thefleet is over30years.Fig.2shows the world-wide distribution of nuclear power plants classified by years of commercial operation[11].Since the original license period in the USA is40years,many reactor opera-tors are seeking license renewal to allow them to operate the plants for an additional20years.To date,73of the 104operating commercial reactors in the USA have received license extensions with another13applications under review,and a key question is how long can these plants be safely,reliably and economically operated.The limiting factor is whether critical materials can continue to maintain their integrity beyond60years[5].These mate-rials include reactor components,concrete,cables and bur-ied piping.So the lifetime of the current reactorfleet is ultimately governed by the performance of materials.1.2.Major materials degradation modes in nuclear energy systemsIn addition to satisfying standard materials design crite-ria based on tensile properties,thermal creep,cyclic fatigue and creep-fatigue,structural materials for current and pro-posed future nuclear energy systems must provide adequate resistance to two additional overarching environmentalThere arefive key bulk radiation degradation effects (low temperature radiation hardening and embrittlement; radiation-induced and-modified solute segregation and phase stability(including amorphization);irradiation creep;void swelling;and high-temperature helium embrit-tlement)[8,12–16],and a multitude of corrosion and stress corrosion cracking effects in water-cooled reactors[13,17–22]and proposed advanced reactors utilizing other cool-ants[23–26](including irradiation-assisted phenomena) that can have a huge impact on the performance of struc-tural materials in nuclear energy systems.The amount of radiation damage produced in materials from exposure to neutrons created by the nuclear energy reactions is quanti-fied by the international standardized parameter[27,28]of displacements per atom(dpa);a displacement damage value of1dpa means that,on average,each atom has been displaced from its lattice site once.Neutron irradiation can produce pronounced hardening at low and intermediate irradiation temperatures due the production of high densities of nanoscale defect clusters (dislocation loops,helium bubbles,etc.),which serve as obstacles to dislocation motion.This hardening is generally accompanied by a reduction in tensile elongation and frac-ture toughness.The radiation hardening and reductions in elongation and fracture toughness typically emerge at dam-age levels above$0.1dpa and are generally most pro-nounced for homologous irradiation temperatures below 0.35T M,where T M is the absolute melting temperature [26,29–35].Fig.3shows an example of the effect of moder-ate neutron displacement damage levels on the engineering stress–strain curve for austenitic stainless steel[36]and a8–9%Cr-tempered martensitic steel[35]at250°C.Both materials exhibit significant radiation-induced increases in yield and ultimate tensile stress,large reductions in elonga-tion(particularly uniform elongation)and decreased strain hardening capacity.The reductions in elongation and strain hardening capacity have been attributed toflow localization(e.g.dislocation channeling)[37–44]and strain hardening exhaustion[29–31]mechanisms.In addition to the decreased elongation,neutron irradiation at low tem-perature also generally produces a decrease in fracture toughness.Fig.4summarizes some of the fracture tough-ness data for Types304and316austenitic stainless steels following irradiation at LWR-relevant conditions near 250–350°C[32,36,45–48].The fracture toughness decreases rapidly with increasing irradiation dose,and approaches a value near50MPa m1/2after5–10dpa.The reduction in fracture toughness can be of particular concern for body-centered cubic materials such as ferritic/martensitic steels if the ductile to brittle transition temperature is shifted to temperatures above cold or warm standby temperatures. The potential for neutron radiation-induced embrittlement of reactor pressure vessel steels has been intensively inves-tigated due to its importance for public safety[49].At intermediate temperatures(homologous tempera-tures>0.3T M),the increased mobility of the radiation defects produces a diverse range of potential microstruc-Age distribution of the world’s commercial nuclear power reactorsDecember2011[11].738S.J.Zinkle,G.S.Was/Acta Materialia61(2013)735–758precipitation in austenitic stainless steel for temperaturesas low as300°C[50].Void swelling(due to nucleation and growth of the supersaturation of vacancies produced by irradiation)is characterized by an initial low-swelling transient regime at low doses(during the void nucleation and initial growth phase),followed by a steady-state swell-ing regime where the volumetric swelling increase is pro-Fig.5.Precipitate phases observed in Type316austenitic stainless steel after neutron irradiation as a function of temperature and dose.Partially shaded data points at temperatures<400°C denote the presence of c phase and solid data points are for either G and related phases or an unidentified phase[50].portional to the dose [12,16,53–55].Typical post-transient steady-state swelling rates in irradiated metals are $0.2–1%dpa À1,which would produce unacceptable volumetric swelling in structural components exposed to high neutron doses.Therefore,research has focused on identifying mech-anisms that extend the low-swelling transient regime and delay the onset of the steady-state swelling regime [55,56].Irradiation creep [12,53,57–60]and irradiation growth [58–61]can cause substantial dimensional changes in addi-tion to changes due to void swelling.Irradiation growth is mainly an issue in anisotropic crystallographic systems,such as hexagonal close-packed materials;for this phenom-enon,volume is conserved but pronounced anisotropic expansion in one crystallographic direction (and shrinkage in another direction)can occur due to preferential nucle-ation of defect clusters,such as dislocation loops,on cer-tain crystallographic habit planes.Materials for nuclear energy systems that exhibit irradiation growth include graphite and pure metals or alloys based on zirconium and beryllium.The amount of deformation from irradia-tion creep is typically proportional to the applied stress and irradiation exposure,with a steady-state creep compli-ance coefficient of 0.5to 1Â10À6MPa À1dpa À1for ferriticing temperature of materials in nuclear energy systems to temperatures significantly lower than what would be estab-lished by thermal creep strength considerations.2.Materials challenges in current commercial fission reactors2.1.Operating environment for materials in existing LWRs Materials in LWRs are exposed to a variety of condi-tions.In the following,the operating environment for nor-mal,extended life and transient conditions are ed fuel disposition issues,while important,are not discussed in this paper.2.1.1.LWR materials under normal operating conditions Core materials include both fuel materials and structural components.The fuel consists of UO 2pellets in the shape of right circular cylinders with length and diameter of approximately 1cm each,loaded into 3–4m long zirco-nium alloy fuel tubes (cladding),which are grouped into fuel assemblies containing control rods or blades.In BWRs,the assemblies generally contain approximately 740S.J.Zinkle,G.S.Was /Acta Materialia 61(2013)735–758control rods are distributed throughout the square lattice and are connected to each other to form a control rod clus-ter,as shown in Fig.8.In both cases,control rods consist of stainless steel tubesfilled with boron carbide for neutron absorption.There is no channel box around a PWR assem-bly,so cross-flow of water between assemblies is possible. The PWR and BWR cores are typically operated nonstop for18–24months between refueling operations.Low and intermediate burn-up fuel assemblies are typically moved to different positions in the core during refueling outages to provide optimized fuel management,with a total core residence period of3–4fuel cycles(i.e.a third to a quarter of the fuel assemblies are removed each refueling cycle) until they achieve typical cumulative burn-up levels of $40–60GW days per metric ton of uranium(GWd MTUÀ1),corresponding tofissions in$4.2–6.4%of the original uranium atoms.Non-fuel core components consist of major structures such as the core shroud(BWR)or the baffle–former assem-bly(PWR),and smaller components such as bolts,springs, support pins and clips.The core shroud in a BWR is a cylindrical barrel,open at both ends,that surrounds the fuel assemblies.Water from the condenser mixes with water recirculated from the core between the shroud near the top of the vessel and is channeled down the annulusic stainless steel or nickel-base nozzles in the reactor head (PWR)or bottom(BWR),and the RPV.The RPV serves as both a pressure barrier and a con-tainment barrier for the radioactivefission products pro-duced during the nuclearfission reaction,and thus plays a key role in reactor safety.The RPV is typically con-structed from carbon and low alloy ferritic steels with1–2%Mn,0.5–1%Ni,$0.5%Mo and0.15–0.4%Si[67], and has a typical wall thickness of$20cm.Older LWR pressure vessels were constructed from rolled plates that were welded to form a large cylinder,whereas newer vessels are formed from ring forgings in order to eliminate welds in the vessel“beltline”region closest to the center of the reac-tor core.The top and bottom heads are usually constructed from low alloy steel forgings,and are welded to the central cylindrical vessel(or bolted and gasketed in the case of the upper head).The internal surface of the RPV is typically clad with5–10mm of an austenitic stainless steel to pro-vide corrosion compatibility with the reactor coolant.Mul-tiple penetrations for coolantflow and instrumentation are made through the pressure vessel.The fast neutronflux is three to four orders of magnitude lower at the RPV com-pared to core internal structures[67],but it is still of suffi-cient intensity to cause radiation hardening,which could lead to fracture toughness embrittlement.It is important to maintain adequate levels of fracture toughness for a wide variety of operational conditions,including normal operation,cold shutdown for refueling and other mainte-nance,and postulated transient accident scenarios such as pressurized thermal shock in PWRs that would introduce cold water into the reactor vessel while the vessel is at oper-ating pressure and temperature(creating large thermal stresses and potential for crack propagation).Materials utilized in LWR cores must withstand simul-taneous application of mechanical stress,neutron irradia-tion and corrosion due to hot water or steam(see rows1 and2of Table3).Temperatures of core components are in the range275–288°C in BWRs and290–320°C in PWRs.The BWR environment is characterized by an elec-trochemical potential(ECP)in the range of150mV rela-tive to the standard hydrogen electrode,or150mV SHE, due to a combination of boiling of the water in the core and radiolysis.PWRs operate at a lower potential (<À500mV SHE)by virtue of the addition of hydrogen at a level of35cc of H2per kg of water($3ppm)to scavenge radiolysis products and lower the corrosion potential. PWR primary water also contains1000ppm B as boric acid(H3BO3)added for reactivity control and2–4ppm Li as LiOH added for pH control.The lower ECP is better for both corrosion and stress corrosion cracking of core materials and is possible in a PWR due to the lack of boil-ing.In addition to controlling pH,boron impacts the for-mation of solid corrosion products(CRUD)and corrosion of fuel cladding,as well as reactivity control of the reactor,and is briefly described in Section2.2.Stresses on fuel and core components come from a variety of sources,including thermal expansion,high velocity waterS.J.Zinkle,G.S.Was/Acta Materialia61(2013)735–758741flow,residual stresses due to welding,and stresses due to radiation-induced volume expansion or distortion.The unique environmental element of a reactor core is radiation.Fission results in several different types of radia-tion that affect materials in different ways.Principal radia-tion types contributing to material degradation are the fission products,neutrons and gamma rays.Fission prod-ucts consist of high-energy($100MeV)elements of sizable mass(generally between90and150atomic mass units)that result directly from thefission process.These elements are created as highly charged ions that deposit their energy within10l m of their origin.As such,except for those that are born within this distance of the fuel pellet surface,fis-sion product damage is confined to the fuel.Thefission process releases both neutrons and gammas.Neutrons are created with an energy of$2MeV and slow down via collisions with the coolant and structural components.Neutrons are the primary source of radiation damage to core materials and fuel cladding and assembly components.Typical displacement damage exposures to the fuel cladding(replaced every$5years)are about 15dpa.The cumulative displacement damage in core inter-nal structures can approach80dpa after40years.The dis-placement damage rate decreases rapidly with increasing distance from the core due to neutron moderation(lower neutron energy resulting from energy loss via collisions with the coolant and core materials);the displacement damage levels in the RPV wall for a PWR are typically $0.05dpa after40years of operation,and the correspond-ing damage in a BWR vessel wall can be up to an order of magnitude lower.This displacement damage can result in significant temperature-and dose-dependent changes to the microstructure(formation of dislocation loops,precip-itate formation/dissolution,void formation,radiation-induced segregation,etc.)[16],which affects mechanical properties(strength/hardness,ductility,fracture toughness and embrittlement,creep,fatigue).When combined with the environment,high temperature and stress,additional modes of degradation occur such as irradiation-assisted stress corrosion cracking,corrosion fatigue and environ-mentally enhanced fracture toughness degradation.The gamma radiationfield is intense and extends through-out the core,aided by(n,c)reactions in structural compo-nents.While atom displacement by gamma rays is of minor consequence,the main importance of gamma rays is in their heating and changes to water chemistry.Gamma heating can elevate temperatures in thicker components close to the fuel (such as baffle–former plates and bolts)by as much as 60°C above the water temperature.Gamma rays also induce radiolysis of the water and create a number of radicals that elevate the corrosion potential in the core.Corrosion poten-tial is the critical element governing stress corrosion cracking of core materials at elevated temperature.Beyond the materials contained within the reactor ves-sel,the major components affected by the water chemistry environment include piping,turbine rotors and blades,the condenser and,in PWRs,the pressurizer and steam gener-ator.Historically,the main materials degradation prob-lems have been intergranular stress corrosion cracking (IGSCC)of BWR piping and of steam generator tubes and in vessel penetrations in PWRs.IGSCC of BWR pipes and steam lines made of304stainless steel was due to a combination of weld-induced residual stresses and sensiti-zation of grain boundaries caused by heat treatment of high carbon steels in the temperature region in which chro-mium carbides formed rapidly on the boundaries,depleting them of chromium and making them susceptible to attack. IGSCC of Alloy600occurred in steam generators,on both the primary and secondary sides,and was driven by a sus-ceptible microstructure and the creation of crevices on the secondary side in which crevice chemistry was favorable for intergranular attack.2.1.2.Life extension and power upratesDue to the initial high capital cost for construction of nuclear power plants relative to the cost of the fuel and other operating expenses,the levelized cost of electricity for LWRs is dominated by the amortized original cost of construction; the annualized costs associated with the fuel and operating and maintenance costs in a new nuclear power plant are esti-mated to contribute about20%of the levelized cost of nuclear electricity[68].This factor,along with the high capac-ity factor of LWRs demonstrated during the past decade,has led to significant interest in extending the operational licenses of nuclear power plants beyond their initial term(typically 40years).Extension of reactor operating licenses for an addi-tional20years means that reactor components will be required to maintain their integrity for a period that is50% longer than the initial40-year license.This increase in opera-tional life introduces a wide range of potential materials aging issues that must be considered as part of the renewal license process[5,69].While the effect of increased irradiation expo-sure is dependent on the component,the increase in operating life by50%means that displacement damage at the bottom of the top guide in a BWR may be>50dpa,while the shroud will acquire a damage level100times lower.High-fluence components such as baffle bolts will reach damage levels exceeding100dpa.Fig.9shows a rough approximation of thefluence(damage)levels associated with various compo-nent failures(top)in both BWRs and PWRs or with micro-structure/property changes(bottom)and the impact of a20-year increase in operating lifetime.Life extension will increase the maximum expected damage level on the components receiving the highestfluence,and it will also elevate the dam-age level on the balance of components proportionately. Additional life extensions beyond20years are also being con-templated and thefluence(damage)level coinciding with three20-year life extensions is shown for comparison.In addition to life extension activities,a second approach to leveraging the existing capital assets of a nuclear power plant is to make modifications to the operating parameters (e.g.coolantflow rate)and/or changes to existing equip-ment(e.g.turbines)that enable high power levels to be achieved.These power uprate requests require detailedS.J.Zinkle,G.S.Was/Acta Materialia61(2013)735–758743。
核能专业英语
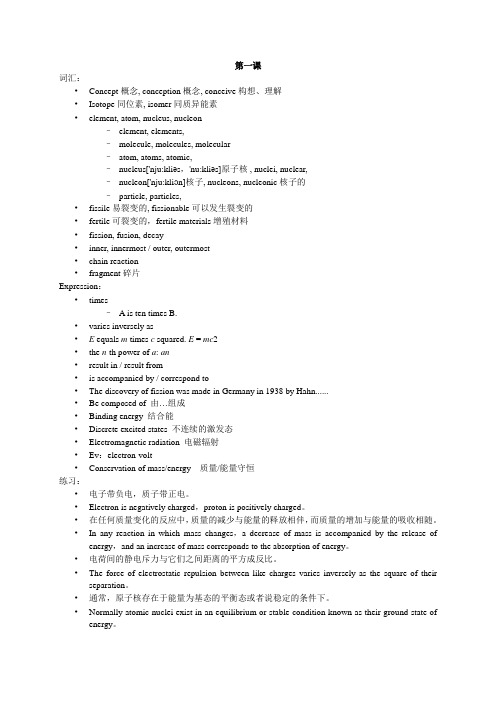
第一课词汇:•Concept概念, conception概念, conceive构想、理解•Isotope同位素, isomer同质异能素•element, atom, nucleus, nucleon–element, elements,–molecule, molecules, molecular–atom, atoms, atomic,–nucleus['nju:kliəs,'nu:kliəs]原子核 , nuclei, nuclear,–nucleon['nju:kliɔn]核子, nucleons, nucleonic核子的–particle, particles,•fissile易裂变的, fissionable可以发生裂变的•fertile可裂变的,fertile materials增殖材料•fission, fusion, decay•inner, innermost / outer, outermost•chain reaction•fragment碎片Expression:•times– A is ten times B.•varies inversely as•E equals m times c squared. E = mc2•the n-th power of a: an•result in / result from•is accompanied by / correspond to•The discovery of fission was made in Germany in 1938 by Hahn......•Be composed of 由…组成•Binding energy 结合能•Discrete excited states 不连续的激发态•Electromagnetic radiation 电磁辐射•Ev:electron-volt•Conservation of mass/energy 质量/能量守恒练习:•电子带负电,质子带正电。
Nuclear Energy and the Environment
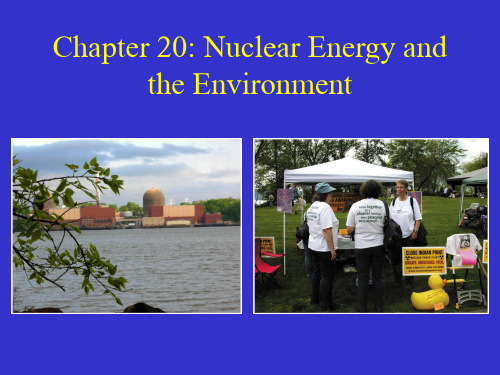
– 2. High density of fuel elements – 3. Plasma must be confined
• Potential energy available if developed nearly inexhaustible.
– Many obstacles remain to be solved
– Two isotopes of hydrogen injected into reactor chamber – Products include helium and neutrons
Fusion Reactors
• Several conditions necessary
– 1. Extremely high temperatures
• Nuclear reactors-devices that produce controlled nuclear fission.
– Used for commercial energy production
Fission Reactors
• First demonstrated in 1942
Radiation Doses and Health
• Determining the point at which the exposure or dose becomes a hazard to health.
– 5,000 mSv is considered lethal in 50% of people – 1,000-2,000 mSv sufficient to cause health problems – 50 msv maximum allowed dose for workers in the industry (30 times ave. natural background)
Carbon Oxidation
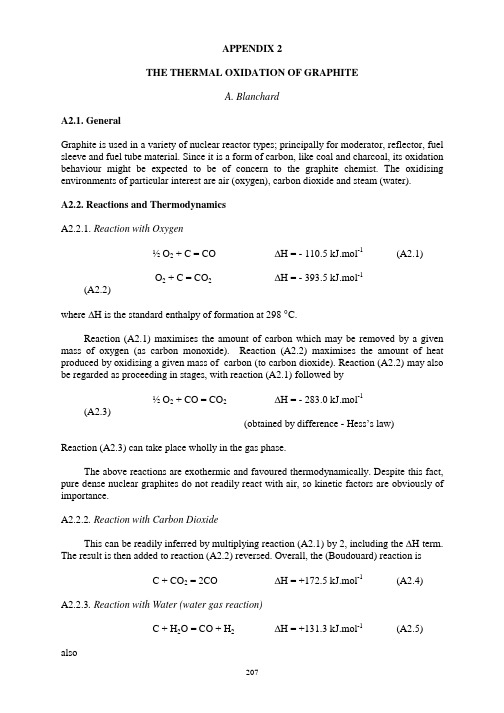
APPENDIX 2THE THERMAL OXIDATION OF GRAPHITEA. BlanchardA2.1. GeneralGraphite is used in a variety of nuclear reactor types; principally for moderator, reflector, fuel sleeve and fuel tube material. Since it is a form of carbon, like coal and charcoal, its oxidation behaviour might be expected to be of concern to the graphite chemist. The oxidising environments of particular interest are air (oxygen), carbon dioxide and steam (water).A2.2. Reactions and ThermodynamicsA2.2.1. Reaction with OxygenO2 + C = CO∆H = - 110.5 kJ.mol-1(A2.1)½O2 + C = CO2∆H = - 393.5 kJ.mol-1(A2.2)where ∆H is the standard enthalpy of formation at 298 °C.Reaction (A2.1) maximises the amount of carbon which may be removed by a given mass of oxygen (as carbon monoxide). Reaction (A2.2) maximises the amount of heat produced by oxidising a given mass of carbon (to carbon dioxide). Reaction (A2.2) may also be regarded as proceeding in stages, with reaction (A2.1) followed by½ O2 + CO = CO2∆H = - 283.0 kJ.mol-1(A2.3)(obtained by difference - Hess’s law)Reaction (A2.3) can take place wholly in the gas phase.The above reactions are exothermic and favoured thermodynamically. Despite this fact, pure dense nuclear graphites do not readily react with air, so kinetic factors are obviously of importance.A2.2.2.Reaction with Carbon DioxideThis can be readily inferred by multiplying reaction (A2.1) by 2, including the ∆H term. The result is then added to reaction (A2.2) reversed. Overall, the (Boudouard) reaction isC + CO2 = 2CO∆H = +172.5 kJ.mol-1 (A2.4)A2.2.3.Reaction with Water (water gas reaction)C + H2O = CO + H2∆H = +131.3 kJ.mol-1 (A2.5) alsoC + 2H2O = CO2 + 2H2∆H = -82.4 kJ.mol-1(A2.6) The hydrogen produced can then react with carbon:C + 2H2 = CH4∆H = -74.81 kJ.mol-1 (A2.7) Subtracting (A2.5) from (A2.6) givesCO + H2O = CO2 + H2∆H = - 213.7 kJ.mol-1(A2.8)This is the water gas shift reaction which takes place in the gas phase.In many practical cases, products from the above reactions are free to escape, such that thermodynamic equilibrium is not reached. The enthalpy changes are of importance, however, since they give a measure of the heat produced in exothermic reactions.A2.3. Mechanisms, Regimes and KineticsA2.3.1. MechanismsThe oxidation mechanisms consist of a series of physical and chemical steps. Non-catalysed oxidation typically follows the route:(i)Transport of oxidant to the graphite surface.(ii)Adsorption of oxidant onto the graphite surface (physisorption).(iii)Formation of carbon-oxygen bonds (chemisorption).(iv)Formation of carbon-hydrogen bonds in reaction (A2.7) (reduction).(v)Breaking of the carbon-carbon bonds.(vi)Desorption of carbon monoxide, or other product.(vii)Transport of reaction product from the graphite surface.Any of the above steps may be rate controlling, i.e. develop the major reactant concentration gradient.A2.3.2.Controlling FactorsFactors controlling the rate of oxidation may include the following:(viii)The rate at which the oxidant is supplied to the surface.(ix)The partial pressure of the oxidant.(x)The reactive surface area available to the oxidant at the surface.(xi)The amount and distribution of catalytic impurities in the graphite.(xii)The temperature.(xiii)The rate at which reaction products are removed.(xiv)The fast neutron damage to the graphite.(xv)The amount of pre-oxidation (radiolytic or thermal burn-off).(xvi)The quantity of in-pore deposits.(xvii)The effective diffusion coefficient.A2.3.3.Oxidation Regimes and KineticsRegime 1At low oxidation rates (generally at relatively low temperatures for the particular oxidation reaction) the oxidant may be at essentially the same concentration throughout the transport pores of the graphite. This ‘chemical’ regime is characterised by the fact that the reaction rate is largely determined by the intrinsic reactivity of the graphite (steps (ii) to (vi), above). Different parts of the structure may react at different rates; the binder being more reactive than the grist particles and edge atoms being more reactive than basal plane atoms, for example. It may also be the case that the gas composition varies in non-transport pores and that the effect of a given gas composition varies with pore shape (because of gas phase reactions).Reaction between air and pure nuclear graphites is generally not measurable below about 350 °C and only becomes significant in the region of 400 °C. The rate of reaction is typically of the order of 3-8 x 10-10 kg.kg-1.s-1 at this temperature (historically expressed in µg/gh; 1µg/gh being 2.78 x 10-10 kg.kg-1.s-1). The chemical regime then extends typically up to 550-600 °C.The units for oxidation rate imply a rate law of the formdm dtk m=(A2.9)wherem = graphite mass (kg)t = time (s)k = a (rate) constant (s-1)However, for a solid reacting body, rate laws of the form shown below would be expected for reaction at the superficial surface (with some simple assumptions):Slabs dmdtk=(A2.10)Cylinders dmdtk m=12(A2.11)Spheres dmdtk m=23(A2.12)The reaction is, of course, taking place within the porous body of the graphite. It cannot, however, be related to the initial open pore volume (which might be expected to be proportional to graphite mass) since the reaction must take place at the surface of the pores. The rate law is thus only superficially similar to a homogeneous first order gas phase expression and care must be taken in its use, particularly in describing the time dependence of graphite burn off.The variation of reaction rate with temperature is of importance:k AeE RT=−(A2.13)whereA is a pre-exponential factor (s-1)E is the apparent temperature coefficient of reaction (‘activation energy’) (J.mol-1)R is the gas constant (J.mol-1.K-1)E has a typical value of 170 kJ.mol-1.Similar considerations apply to reaction with carbon dioxide and water vapour (H2O). The reaction with CO2 is of less importance, however, since it is negligible at 625 °C (Thurlbeck, 1962) and does not pose a problem even at the highest AGR inner sleeve temperatures of 675 °C (Prince, 1976). The reaction is also endothermic (equation (A2.4)) and so does not have the same safety implications as the reaction with air.The reaction with H2O is of particular importance in HTR reactors, because of the (generally small) inleakage from the steam side into the gas circuit, where it can react with hot graphite fuel tubes. Since the partial pressure of the water vapour is a variable in this system, rate equations of the formr A P n=(A2.14)are applied, wherer = the specific reaction rate (kg.kg-1.s-1)A = a (rate) constant (s-1.(N.m-2)-1)P = partial pressure of water vapour (N.m-2)The reaction with water vapour is generally insignificant below 800 °C and approximately obeys equation (A2.14) with n=0.5 over the temperature range 1000-1200 °C. The kinetics can also be described by a Langmuir-Hinshelwood scheme (Walker et al, 1959; Atkins, 1987; Stairmand, 1990).Regime 2In this regime, the reaction rate becomes high enough for access of the gas to the in-porestructure to be significantly limited by diffusion control (steps (i) and (vii), above). This can also arise from a particularly restrictive structure. For the air-graphite system, this occurs approximately in the range 600-900 °C. The ‘activation energy’ is halved in this regime and the kinetic expressions involve the effective diffusion coefficient for the graphite (Walker et al, 1959; Giberson and Walker, 1965).Regime 3This is the mass transfer regime (Burnette et al, 1979; Raeder and Gulden, 1989), where reaction at the superficial surface of the graphite is so high that most of the oxidant is consumed there, the oxidant concentration gradient generally developing across the laminar sub-layer. The reaction rate is now expressed in terms of the superficial surface area of the graphite (kg.m-2.s-1) together with any oxidant partial pressure dependence. The change from one regime to another may be progressive and mode 2 may appear to be missing in some cases.Two other ‘regimes’ appear trivial, but can usefully be distinguished:Regime 4If there is a fixed rate of ingress of oxidant to the system, for example as a known quantity of impurity in the make-up gas, the rate of oxidation cannot exceed the rate of supply of oxidant (rate balance). The preferred site of any resulting oxidation may not be known, however.Regime 5If the system contains a fixed amount of oxidant, for example that remaining after blowing down and recharging the coolant gas, the extent of oxidation is limited by the amount of oxidant available (mass balance). Neither the location, nor the rate of reaction, may be known in this case.A2.3.4.CatalysisThe kinetics in the chemical regime may be further complicated by catalysis (McKee, 1981). The catalyst (impurity) particles act to increase the reaction rate by offering an alternative reaction pathway. This lowers the activation energy. Reaction rates are particularly increased at lower temperatures (this leads to a ‘compensation’ effect).A simple catalytic model involves the oxidation of metal atoms (M) by oxygen, followed by reduction of the oxide by carbon:½ O2 + M = MO(A2.15)MO + C = CO + M(A2.16)The reaction may proceed by a ‘tunnelling’ mechanism.Reaction inhibitors are also known (McKee, 1991) and some substances are able to act to either promote or inhibit reaction, perhaps by competing for active sites (e.g. water/oxygen). Boron (with phosphorus) is known as an inhibitor for thermal oxidation, but when intercalated into the graphite structure will promote oxidation (Karra et al, 1995)(perhaps by mimicking fast neutron damage).A2.4. Requirements for the InformationEarly designs of graphite moderated reactor operated with air as the coolant and there was a requirement to understand both the likely ongoing oxidation behaviour of the graphite and its behaviour during a temperature excursion. As these reactors were superseded by carbon dioxide cooled designs, an understanding of the reaction of graphite with air remained important for the following reasons:(i)Safety case information relevant to both major and minor ingresses of air to the system under fault or other conditions (Dodson, 1960; Nairn and Wilkinson, 1960; Blanchard and Fitzgerald, 1978).(ii)The possible requirements to carry out deliberate oxidations to(a)Remove deposits from fuel.(b)Open up the structure of low diffusivity graphite to improve inhibitor (e.g. methane) access.(c)Remove deposits from fuel pin and heat exchanger surfaces to improve heat transfer.(d)Estimate the amount of deposit in graphite moderator and fuel sleeves by differential thermal oxidation (Welch, 1972; Oxley and Dymond, 1972; Baguley and Livesey, 1972) (so as to be able to correct the weight loss).(e)Alter the structure of experimental graphites in controlled ways to improve theoretical knowledge of the interaction between reactivity and structure.A2.5. Essential Measurements and KnowledgeThe complexity of graphite oxidation behaviour is such that the following are generally required:(i)Good statistical data on the relevant oxidation rates for a range of blocks and heats. (ii)An awareness of the relevant oxidation regime and the rate laws which are likely to apply.(iii)Information on the temperature coefficient of reaction.(iv)Information or measurement on the heat change on reaction.(v)Theoretical or experimental information on factors affecting the oxidation rate, such as fast neutron damage, burn-up, deposition of potential catalysts, etc.(vi)Modelling knowledge to extrapolate from small scale samples, or full scale tests.As some graphite reactors are coming to the end of their lives, there is an increasing requirement to carry out assessments for long term storage or disposal (Wickham et al, 1996). The potential oxidation behaviour is also of concern in this context.A2.6. AcknowledgementsIn compiling this account, the author has been particularly grateful for reviews by John Stairmand (Stairmand, 1990) and Tony Wickham et al (Wickham et al, 1996) who in turn cite the pioneering work of P.L. Walker (Walker et al, 1959) and Dubinin (Dubinin, 1966). Any important omissions are the author’s own, however.REFERENCES[1]Atkins P.W. Physical Chemistry, 3rd edition, Oxford University Press, 783 (1987).[2]Baguley G. and Livesey J.N. DTO Applied to Graphite/Carbon Deposit Systems - Paper11, The Attack Rate of the underlying Graphite during Oxidation of In-pore Deposits,Symposium on Differential Thermal Oxidation held at Berkeley Nuclear Laboratories (1972).[3]Blanchard A. and Fitzgerald B. The Air Reactivity of WAGR Moderator and SleeveGraphites, UKAEA Report WAGR/TC/P(78)21 (1978).[4]Burnette R.D., Velasquez C. and Harrington R.O. Studies of the Rate of Steam Oxidationof Graphite at High Gas Velocity, 14th Biennial Conference on Carbon, Pennsylvania State University (1979).[5]Dodson M.H. The Depth of Oxidation of Graphite: A Theoretical Approach, UKAEAReport DEG 148 (CA) (1960).[6]Dubinin M. Chemistry and Physics of Carbon, 2 (Ed. P.L. Walker, Jr), Marcel Dekker,NewYork, 51 (1966).[7]Giberson R.C. and Walker J.P. Reaction of Nuclear Graphite with Water Vapour - Part1:Effect of Hydrogen and Water Vapour Partial Pressures, Battelle Northwest Report, BNSA-181 (1965).[8]Karra M., Zaldivar R.J, Rellick G.S., Thrower P.A. and Radovic L.R. SubstitutionalBoron in Carbon Oxidation - Inhibitor or Catalyst?, Proc. 22nd Biennial Conference on Carbon, San Diego (1995).[9]McKee D.W. Chemistry and Physics of Carbon, 16 (Ed. P.L Walker, Jr), Marcel Dekker,New York, 1 (1981).[10]McKee D.W. Chemistry and Physics of Carbon, 23 (Ed. P.L Walker, Jr), Marcel Dekker,New York, 173 (1991).[11]Nairn J.S. and Wilkinson V.J. The Prediction of Conditions for Self-sustaining GraphiteCombustion in Air, Paper GCM/UK/11, US/UK Compatibility Conference (1960). [12]Oxley E. and Dymond B. DTO Applied to Graphite/Carbon Deposit Systems - Paper 8,DTO Studies of Irradiated Graphite using Gas Chromatography, Symposium onDifferential Thermal Oxidation held at Berkeley Nuclear Laboratories (1972).[13]Prince N. Experience in the Design of Graphite Moderator Structures, Paper presented tothe Nuclear Engineering Society (1976).[14]Raeder J. and Gulden W. Safety Analyses performed by NET, IAEA TechnicalCommittee Meeting on Fusion Reactor Safety, Jackson, Wyoming (1989).[15]Stairmand J.W. Graphite Oxidation - A Literature Survey, AEA Technology ReportAEA-FUS-83 (1990).[16]Thurlbeck A. Summarised Compatibility Review of Reactor Materials for CO2 -cooledGraphite Moderated Reactors, TRG Report 267(W) (1962).[17]Walker P.L., Rusinko F. and Austin L.G. Advances in Catalysis, 11, 123 (1959).[18]Welch E.E. DTO Applied to Graphite/Carbon Deposit Systems - Paper 1, Principles andProblems, Symposium on Differential Thermal Oxidation held at Berkeley NuclearLaboratories (1972).[19]Wickham A.J., Marsden B.J., Pilkington N.J. and Heath T.G. The Possibility andConsequences of Graphite Core Degradation during ‘Care and Maintenance’ and‘Safestore’, AEA Technology Report AEA/16423595/R/001 (1996).。
能源环境双语15题(附答案)
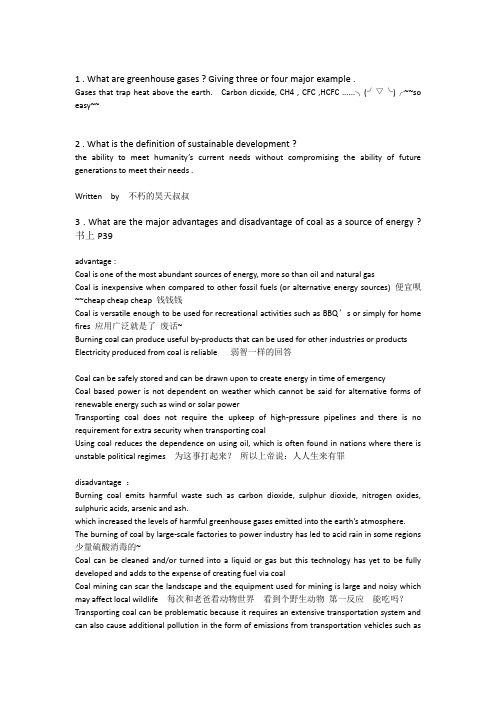
1 . What are greenhouse gases ? Giving three or four major example .Gases that trap heat above the earth. Carbon dicxide, CH4 , CFC ,HCFC ......╮(╯▽╰)╭~~so easy~~2 . What is the definition of sustainable development ?the ability to meet humanity’s current needs without compromising the ability of future generations to meet their needs .Written by 不朽的昊天叔叔3 . What are the major advantages and disadvantage of coal as a source of energy ? 书上P39advantage :Coal is one of the most abundant sources of energy, more so than oil and natural gasCoal is inexpensive when compared to other fossil fuels (or alternative energy sources) 便宜呗~~cheap cheap cheap 钱钱钱Coal is versatile enough to be used for recreational activities such as BBQ’s or simply for home fires 应用广泛就是了废话~Burning coal can produce useful by-products that can be used for other industries or products Electricity produced from coal is reliable 弱智一样的回答Coal can be safely stored and can be drawn upon to create energy in time of emergencyCoal based power is not dependent on weather which cannot be said for alternative forms of renewable energy such as wind or solar powerTransporting coal does not require the upkeep of high-pressure pipelines and there is no requirement for extra security when transporting coalUsing coal reduces the dependence on using oil, which is often found in nations where there is unstable political regimes 为这事打起来?所以上帝说:人人生来有罪disadvantage :Burning coal emits harmful waste such as carbon dioxide, sulphur dioxide, nitrogen oxides, sulphuric acids, arsenic and ash.which increased the levels of harmful greenhouse gases emitted into the earth’s atmosphere. The burning of coal by large-scale factories to power industry has led to acid rain in some regions 少量硫酸消毒的~Coal can be cleaned and/or turned into a liquid or gas but this technology has yet to be fully developed and adds to the expense of creating fuel via coalCoal mining can scar the landscape and the equipment used for mining is large and noisy which may affect local wildlife 每次和老爸看动物世界看到个野生动物第一反应能吃吗?Transporting coal can be problematic because it requires an extensive transportation system and can also cause additional pollution in the form of emissions from transportation vehicles such aslorries, etc 还是污染~前面都说了~ 所以说老师的ppt有3/2是废话~There are limited stocks of coal remaining - they will be entirely depleted this millennium if we continue to burn coal in the future at the same rate we are today. Coal is considered as a non-renewable energy sourceThe mining industry can cause health difficulties for miners and fatalities due to the potentially dangerous nature of the work 拯救矿工运动可以了挑几个写写意思意思~~Written by 不朽的昊天叔叔4 . What are some possible solutions to the problem of the detrimental effects of acid rain ? p232 少烧煤炭烧了之后烟气脱硫5 . what is the process called by which an atom can release energy which is then captured and used by a nuclear reactor for power production ?Nuclear Fission 裂变嘛~~ Fission chain reaction 更加地道~以下是易混淆的:热核反应thermonuclear reaction连锁反应chain reaction;chain-reactingripple;effect聚变反应Fusion裂变反应Fission衰变反应Decay裂解反应fragmentation reaction;scission reaction6 . what are rhe major hazards of nuclear power ?Unresolved high level radioactive waste problemPoor technology, operation (human error), and plant design can lead to costly and deadly accidents (e.g., Chernobyl)切尔诺贝利乌克兰基辅边上啊世界上美女质量最高的地方(我甚至怀疑~这事故真的是坏事?)这也是哥学俄语的重要原因之一你懂得Written by 不朽的昊天叔叔7 . How does the fusion process produse energy ?In a fusion reactor, hydrogen (H) atoms come together to form helium (He) atoms, neutrons (中子) and vast amounts of energy.8 . Giving the main types of solar thermal electricty . 光电利用,热能利用说的都要吐了Direct solar energy :architectural designs use solar energy to heat and cool homes(呵呵尽管问的是发电),solar panels used to heat water to drive turbine (这词的读法是“two白因”不是“透平”)Indirect solar energy:heat from solar energy drives other Earth systems which can be harnessed ,Moving water - hydroelectric power ,Biomass ,Wind (然后发电)Putting Solar Energy to Use: Solar Cell/Panel ~~~~~~~~~~~~~~~ Photovoltaic ElectricitySolar Thermal Electricity:Trough systems (槽式系统)Solar Thermal Electricity: Solar Dish/Engines(碟式太阳能发电)Written by 不朽的昊天叔叔9 . what are the major advantages and disadantages of wind energy ? 书上p94 advantage:Wind energy is an economical power resource. ?Wind is a clean fuel;wind farms produce no air or water pollution because no fuel is burned.disadantage:风力发电在生态上的问题是可能干扰鸟类,如美国堪萨斯州的松鸡在风车出现之后已渐渐消失(松鸡会飞?)许多地区的风力有间歇性intermittent进行风力发电时,风力发电机会发出庞大的噪音,所以要找一些空旷的地方来兴建。
rohm Sensor types 数据手册
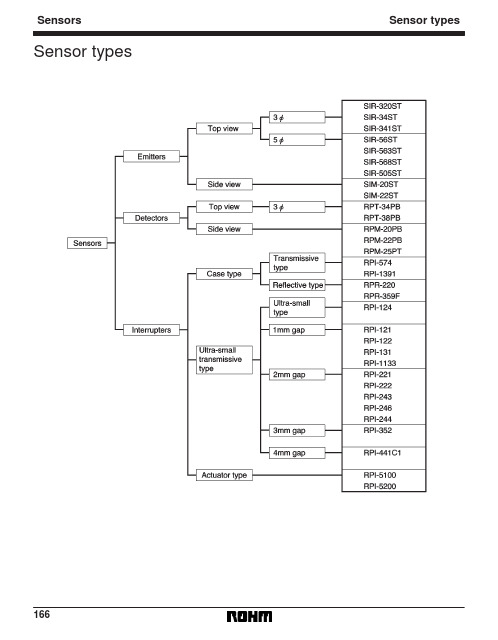
Sensors
Sensor types
Sensor types
166Leabharlann AppendixNotes
No technical content pages of this document may be reproduced in any form or transmitted by any means without prior permission of ROHM CO.,LTD. The contents described herein are subject to change without notice. The specifications for the product described in this document are for reference only. Upon actual use, therefore, please request that specifications to be separately delivered. Application circuit diagrams and circuit constants contained herein are shown as examples of standard use and operation. Please pay careful attention to the peripheral conditions when designing circuits and deciding upon circuit constants in the set. Any data, including, but not limited to application circuit diagrams information, described herein are intended only as illustrations of such devices and not as the specifications for such devices. ROHM CO.,LTD. disclaims any warranty that any use of such devices shall be free from infringement of any third party's intellectual property rights or other proprietary rights, and further, assumes no liability of whatsoever nature in the event of any such infringement, or arising from or connected with or related to the use of such devices. Upon the sale of any such devices, other than for buyer's right to use such devices itself, resell or otherwise dispose of the same, no express or implied right or license to practice or commercially exploit any intellectual property rights or other proprietary rights owned or controlled by ROHM CO., LTD. is granted to any such buyer. Products listed in this document use silicon as a basic material. Products listed in this document are no antiradiation design.
NUCLEARWASTE
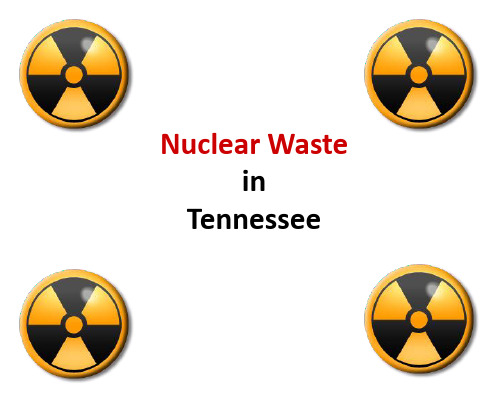
Nuclear Wastein TennesseeTN’s Leadership Role as Radioactive Waste Disposer for the NationIn the year 2000 (the last year that the NRC compiled these numbers in a state by state comparison) Tennessee sent 58.6% of the materials that were disposed at the 3 landfills in the U.S. licensed to receive radioactive materials . Add the radioactive materials that were incinerated and land -filled and Tennessee received at least 75% of the nation’s low-level radioactive waste.HOW AND WHY ARE WEGETTING“LOW-LEVEL” RADIOACTIVE WASTE IN OUR SOLID WASTE LANDFILLS?The N-Waste Disposal•Many oldernuclear reactorsand weaponsfacilities havebeen closed.•The home stateswant to get rid oftheir waste.•Tennessee, the VOLUNTEER state, has licensed nuclear processors that reduce the bulk of waste,but not its radiation.BSFR Program is Born Decades ago TDEC entered into agreements with the nuclear industry to create the Bulk Survey For Release program. (Note that the name does not include the words nuclear or radioactive.).TDEC’s SECRECYTDEC held no public hearings to inform the people, local officials, or the TN legislature of their nuclear agreement. The BSFR program would still be secret if the national nuclear watchdog NIRS had not reported it, and then it was publicized in news stories on Channel 4 television in Nashville.SPEEDY PROCESSINGUnlike the NRC and other states, TNdoes not analyze incoming waste on a case-by-case basis. TDEC stands alone among the state regulators inthe extent to which they havereduced the "regulatory burden"on companies that produce, processand/or dispose of radioactive waste.How does Tennessee Department of Environment & Conservation Measure & Monitor Radiation Levels in Tennessee’s Landfills?ANSWER: BY TRUSTsource TDEC•Processors bringing Radioactive Waste into Tennessee are responsible for monitoring the levels of radiation from point of source—i.e. self-monitoring •Private processors decide which nuclear materials can be reclassified from licensed radioactive waste to regular trash that goes to our municipal landfills—more self-monitoring. TDEC occasionally inspects the paper work.Where does Rad Waste come from?•Only 20%of the low level radioactive waste that goes into TN landfills is generated from within the state.•Waste from decommissioned reactors and other sources comes to TN for processing and burial from as far away as California, Michigan, Connecticut, Washington state, New York and others. Some has come from Canada, Mexico and Brazil, perhaps other countries.The state receives 1 ½ cents per pound for all radioactive waste that goes to processors.AMOUNTS OF Rad Waste in 2004-2006 38,343,961 lbs in 3 years2004•North Shelby 3,677,876•South Shelby 0•Carter Valley 0•Chestnut Ridge 0•Middle Point (fromImpact 165,858)2005•North Shelby 9,823,073•South Shelby o •Carter Valley 7,481,581•Middle Point (Impact 10,130,000)2006•North Shelby 1,302,663•South Shelby 966,937•Carter Valley 3,261,010•Chestnut Ridge 191,194•Middle Point 1,343,769 (Impact 590,570; Toxco 753,199)POUNDS of Rad Waste 2007-09 Landfill 2007 2008 2009North Shelby 2,000,000 180,000 179,000 South Shelby 530,000 850,000 1,770,000 Middle Point 400,000 0 0 Chestnut Ridge 1,300,000 890,000 1,861,000 Carters Valley 495,000 140,000 150,000_________ _______ ________4,725,000 2,060,000 3,960,000 TOTAL FOR 3 YRS: 10,745,000 poundsShelby County•North Shelby County Landfill has received the most radioactive waste of any landfill in TN, over 17 Million Pounds in 6 years.•The city of Memphis depends upon an underground aquifer for its drinking water.•If the drinking water is contaminated, the health and welfare of 670,000 people will be threatened. Memphis is the largest city in Tennessee.•Memphis lies on an earthquake fault. Even a minor earthquake might damage those landfills and pollute the water.Memphis AquiferTypes of Radioactive WasteHigh-Level waste does not go into landfills. It sits in casks and cooling pools on the reactor sites.The U.S. has no middle level classification. What is classified as Mid-Level waste in Europe is called “Low-Level” in the U.S.“Low-Level” Radioactive Waste does not mean “low risk.” “Low-level” waste includes the same atoms as high level waste--plutonium, cesium, strontium, and iodine--but in lower concentrations.Kinds of Low-Level WasteClass A—lowest level Class B—higher level Class C—highest level Greater that Class CLandfill Facts •Municipal landfills were never intended for radioactive waste•LINER LIFE IS 30 YEARS; radiation lasts for thousands of years or more.•Eventually most if not all landfills leak.•Once radioactive material is buried, the state does no further checking on thecontents. Leachate is not checked forradiation.Logic would suggest that if you keep adding more of something to a heap, the total amount would become greater. According to TDEC, this logic does not apply to radioactive waste. No matter how much is added, it all amounts to 1 millirem of exposure per year, forever and ever.The Fantasy of RESRADMANRESRAD is a computer model designed to estimate radiation doses and risks from RESidual RADioactive materials.REALITY--Middle Point Landfill in Walter Hill, Tennessee, on Stones RiverMiddle Point and Water PlantDANGERS of Radioactive Wastesources: EPA & physicians •INGESTED RADIATION, whether breathed in from the air, or consumed through drinking water, or from foods grown on contaminated land, is far more toxic to living creatures than external radiation.•Radiation ACCUMULATES in the body. The doses we take in, whether from natural or man-made sources, accumulate over our lifetimes and damage our cells. Cancer can take 50 years to develop, or much less.There is no such thing as a safe dose, no matter how small.BEIR VII REPORT of the National Academy of Sciences: Study of the health risks from exposure to low levels ofIonizing Radiation•The committee concludes that the higher thedose, the greater the risk.•In case of exposure of fetuses in the womb,increased incidences of cancer can be detectedat low doses.•Cells do not necessarily have to be hit directlyby a radiation track for the cell to be affected,damaging the cell’s DNA.•The committee concludes that thepreponderance of information indicates thatthere will be some risk, even at low doses.ANOTHER EXPERT OPINION “There is no safe level of exposure and there is no dose of radiation so low that the risk of a malignancy is zero.”--Dr. Karl Morgan, from Oak Ridge, dubbed the father of Health PhysicsTN Radioactive Waste Facilities •EnergySolutions at Oak Ridge and Memphis•Studsvikand StudsvikRACE at Erwin and Memphis•Impact at Oak Ridge•PermaFix at Kingston and Oak Ridge •Nuclear Fuel Services at Erwin •Philotechnics at Oak Ridge •Bionomics at Oak Ridge•Aerojet at JonesboroughMany of the 104 operating US nuclear power reactors are now contracted to send their nuclear waste to Studsvik in Erwin &/or Memphis.Processors in TN that Heat TreatRadioactive Waste •EnergySolutions in Oak Ridge-2 incinerators•DSSI PermaFix in Kingston-radioactive and mixed waste boiler, processes PCBs•Studsvik in Erwin--pyroprocessing•IMPACT in Oak Ridge –pyroprocessing•Duratek metal melt in Oak Ridge•TOXCO metal melt in Oak Ridge•Aerojet oxidizer in Jonesborough (metallic uranium chips)IMPORTATION OF FOREIGN WASTE •ENERGY SOLUTIONS wants to IMPORT 1,000 tons of radioactive waste from Germany to burn inOak Ridge. This will open the door to Europe’s“LOW LEVEL” RADIOACTIVE WASTE coming toour state.•ENERGY SOLUTIONS withdrew its application to import 20,000 tons of rad waste from Italy, but it is reworking the application. The original planwas to burn, melt and otherwise process inTennessee these materials from decommissioned nuclear plants.ABOUT INCINERATORS1)Older incinerators tend to be more pollutingthan are newer ones that have moreprocesses for cleaning out pollutants.The EnergySolutions incinerators that willburn German waste are over 20 years old. 2) When incinerators stop and start up again,they emit large amounts of dioxin, a potent carcinogen.EnergySolutions says they will clean out their incinerators before and after each burn ofGerman waste.Facts about Incineration •Incineration produces toxic and carcinogenic substances such as dioxins, furans, particlate matter, heavy metals such as lead and mercury.•When considering the impact of an incinerator, it is necessary to consider the collective impact of incinerators in the region.•Oak Ridge has 4 incinerators within fairly close proximity.•To our knowledge, NO environmental impact study has been ever been done.The incinerators at Oak Ridge have burnt radioactive waste since the late 1980s. Small particles can travel great distances. Of the 25 cities in the U.S. most polluted by Year-Round Particle Pollution, Knoxville-Sevierville-LaFollette, TN, ranked 21st in the nation in 2010. (source: American Lung Association)For other facts on incineration see printed “Incineration: A Factsheet “Bringing Higher Level Waste toTennesseeTwo Tennessee processors want to bring higher level Class B and C radioactive waste to TN from nuclear reactors in 36 states.EnergySolutions would lower the classification by blending with less concentrated Class A waste in Oak Ridge.Studsvik would cook these radioactive resins for significant volume reduction in Erwin, TN.ErwinErwin, TN, is home to both Nuclear Fuel Services and Studsvik.A study released on Nov. 11, 2010, shows the troubled Nuclear Fuel Services in Erwin is apparently discharging enriched uranium into the Nolichucky River. Uranium was found up to 45 miles down river from the plant.PRESIDENT’S ISLAND IN MEMPHISRADIOACTIVE STEAM GENERATORS The Studsvik facility on President’s Island in Memphis is the only place in the U.S. where radioactive steam generators from pressurized water reactors are taken for processing or dismantling. These units are up to 70 feet tall and weigh as much as 800 tons. These must be taken apart, piece by piece to separate the parts that are highly radioactive. These contain a significant amount of plutonium and other dangerous radionuclides. Much of the material deemed to be “extremely low level” by Studsvik will end up in the North or South Shelby landfills.Intestines of Steam Generators that go to MemphisFEDERAL EEOC VIOLATIONS RACE (Radiological Assistance Consulting and Engineering) in Memphis, purchased by Studsvik, agreed in January of 2010 to pay a $650,000 fine to settle claims by the Federal Equal Employment Opportunity Commission. It had intentionally exposed African-American employees to higher levels of radiation than those faced by white workers. TDEC has taken NO ACTION.PUBLIC PARTICIPATION•The nuclear industry in Tennessee has been a high risk enterprise with low visibility.•The Secret City has spawned a secret industry.•The Division of Radiological Health has no control board and INADEQUATE OVERSIGHT.•The Division of Radiological Health does not allow meaningful public participation.•The DRH needs to make documentsavailable to the public in electronic form.Their website is inadequate.Editorial Cartoon: Nuclear WasteYou can’t see it, smell it, taste it, or feel it. Clay Bennett, Washington Post Writers Group, April 1, 2010。
Thenuclearfuelcycle
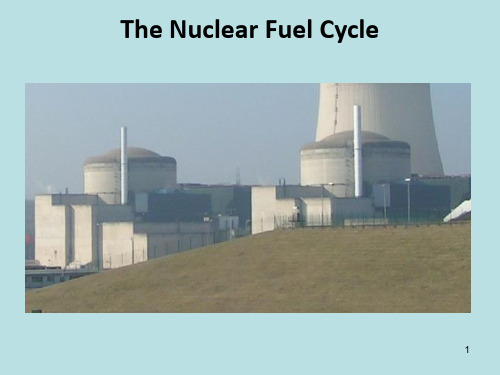
• FRONT END: the preparation of the fuel, steps in the service period in which the fuel is used during reactor operation, and
• BACK END: safely manage, contain, and either reprocess or dispose of spent nuclear fuel.
• An increasing proportion of the world's uranium now comes from in situ leaching (ISL), where oxygenated groundwater is circulated through a very porous orebody to dissolve the uranium and bring it to the surface. ISL may be with slightly acid or with alkaline solutions to keep the uranium in solution. The uranium is then recovered from the solution as in a conventional mill.
- 1、下载文档前请自行甄别文档内容的完整性,平台不提供额外的编辑、内容补充、找答案等附加服务。
- 2、"仅部分预览"的文档,不可在线预览部分如存在完整性等问题,可反馈申请退款(可完整预览的文档不适用该条件!)。
- 3、如文档侵犯您的权益,请联系客服反馈,我们会尽快为您处理(人工客服工作时间:9:00-18:30)。
D Turbine D ==Turbine Turbine D = Turbine DD==Turbine Condenser EE= Condenser Condenser E ==Condenser EE==Condenser Containment Structure FF= Containment Structure Containment Structure F ==Containment Structure FF==Containment Structure
TYPES OF NUCLEAR REACTORS
Coolant. A liquid or gas circulating through the core so as to transfer the heat from it. Steam generator. (not in BWR) Part of the cooling system where the primary coolant bringing heat from the reactor is used to make steam for the turbine. Containment. The structure around the reactor core. It is typically a metre-thick concrete and steel structure.
G Electric Generator G ==Electric Generator Electric Generator G ==Electric Generator GG=Electric Generator H To Power Grid H ==To Power Grid To Power Grid H ==To Power Grid HH=To Power Grid Pump PP= Pump Pump P ==Pump PP==Pump
C F Bຫໍສະໝຸດ GD HLarge body of water
A
E
FEATURES COMMON TO MOST NUCLEAR REACTORS
Over 16% of the world’s electricity is produced from nuclear energy. Several designs for nuclear power reactors have been successfully developed, but there are some features common to most reactors: Fuel - Uranium is the basic fuel. Usually pellets of uranium oxide (UO2) are arranged in tubes to form fuel rods. Moderator - This is material in the core which slows down the neutrons released from fission. Commonly water, but can also be heavy water or graphite. Control rods - These are made with neutron-absorbing material such as cadmium, hafnium or boron, and are inserted or withdrawn from the core to control the rate of reaction
Diagram of a Pressurized Water Reactor (PWR)
A Reactor Core A ==Reactor Core Reactor Core A ==Reactor Core AA=Reactor Core B Control rods B ==Control rods Control rods B ==Control rods BB=Control rods Steam Generator CC= Steam Generator Steam Generator C == Steam Generator CC== Steam Generator