Laboratory drying of organic-matter rich soils- Phosphorus solubility
土壤饱和含水率英文
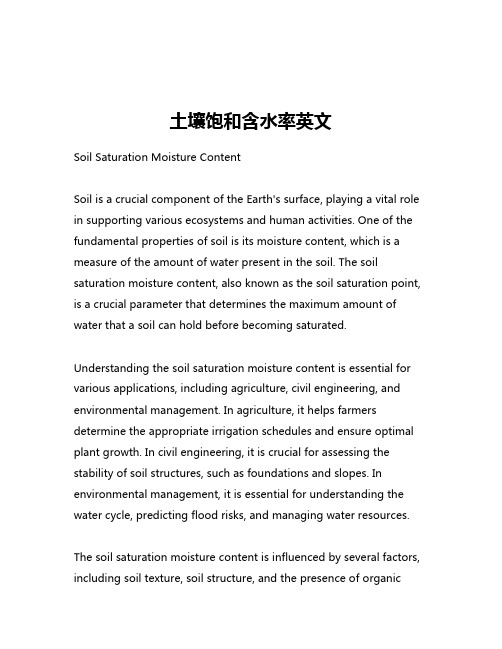
土壤饱和含水率英文Soil Saturation Moisture ContentSoil is a crucial component of the Earth's surface, playing a vital role in supporting various ecosystems and human activities. One of the fundamental properties of soil is its moisture content, which is a measure of the amount of water present in the soil. The soil saturation moisture content, also known as the soil saturation point, is a crucial parameter that determines the maximum amount of water that a soil can hold before becoming saturated.Understanding the soil saturation moisture content is essential for various applications, including agriculture, civil engineering, and environmental management. In agriculture, it helps farmers determine the appropriate irrigation schedules and ensure optimal plant growth. In civil engineering, it is crucial for assessing the stability of soil structures, such as foundations and slopes. In environmental management, it is essential for understanding the water cycle, predicting flood risks, and managing water resources.The soil saturation moisture content is influenced by several factors, including soil texture, soil structure, and the presence of organicmatter. Soil texture refers to the relative proportions of sand, silt, and clay particles in the soil. Sandy soils, for example, have larger pore spaces and tend to have a lower saturation moisture content compared to clay soils, which have smaller pore spaces and can hold more water.Soil structure, on the other hand, refers to the arrangement of soil particles and the formation of aggregates. Well-structured soils with good aggregation tend to have a higher saturation moisture content due to the presence of larger pore spaces. Organic matter in the soil also plays a crucial role in increasing the soil's water-holding capacity, as it can absorb and retain moisture more effectively than mineral soil particles.To measure the soil saturation moisture content, various methods can be used, including laboratory analysis, field measurements, and remote sensing techniques. Laboratory analysis involves taking soil samples and determining the moisture content using gravimetric or volumetric methods. Field measurements can be performed using soil moisture sensors, which measure the electrical conductivity or dielectric properties of the soil to estimate the moisture content.Remote sensing techniques, such as satellite or aerial imagery, can also be used to estimate the soil moisture content over larger areas. These methods rely on the relationship between the soil's reflectanceor emissivity and its moisture content, and they can provide valuable information for large-scale applications, such as regional water management or drought monitoring.Once the soil saturation moisture content is known, it can be used to inform various decisions and management practices. In agriculture, the information can be used to optimize irrigation schedules, reduce water waste, and improve crop yields. In civil engineering, it can be used to assess the stability of soil structures and inform the design of foundations, slopes, and other infrastructure.In environmental management, the soil saturation moisture content is crucial for understanding the water cycle and predicting the occurrence of floods and droughts. It can also be used to inform the management of water resources, such as the allocation of water for different uses and the implementation of conservation measures.In conclusion, the soil saturation moisture content is a fundamental property of soil that has far-reaching implications for various applications. Understanding and accurately measuring this parameter is essential for effective soil and water management, as well as for addressing the challenges posed by climate change and other environmental factors. By leveraging the knowledge and tools available, we can better manage our soil and water resources, ensuring their sustainability for generations to come.。
soil organic matter
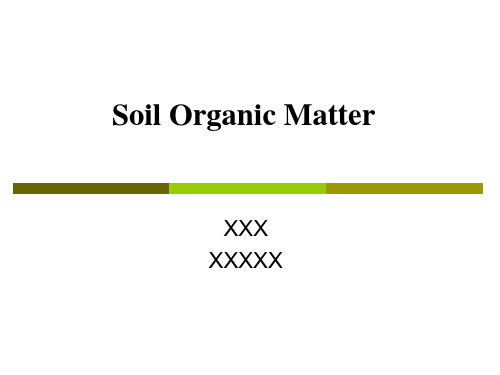
soil texture
Sources
Litter Mainly plant debris and metabolites,animal carcass and excreta Leachates Throughfall and stemflow containing nutrient ions and soluble organic compounds
• The vulnerability of SOM to degradation will depend on the nature of the disturbance as well as the stabilization and destabilization mechanisms at play in a given ecosystem( Schmidt M W I, 2011)
plant debris and animal carcass Microbial remains
Litter
Soil development
Decomposition and transformation
Transformation
Transformation of SOM witl eight insights, contrasting historical and emerging views of soil carbon cycling(Schmidt M W I, 2011)
•
Traditional and emergent views of the nature of soil organicmatter affect how we predict and manage soil, air and water. (Lehmann J and
英语摘要写法

摘要(Abstract)摘要(Abstract) 也成为内容提要,通常在学士论文中都必须附有摘要,其位置应放在论文的正文之前,对整个论文内容的概述。
无论对专业读者还是对非专业读者而言,摘要都是一个非常重要的文件。
摘要如果和论文一起发表,则被称为一次性出版物摘要,主要用于帮助读者评价文章内容及其潜在作用,使读者不必阅读全文就可以了解论文的内容。
除此之外,摘要也可以被单独收入文摘机构出版的摘要期刊如:生物学文摘(Biological Abstract)、化学文摘(Chemical Abstract)等、称为二次性出版物摘要。
此类脱离论文独立成篇的摘要主要用于方便读者检索文献、收集信息,帮助研究者寻找新的研究领域。
一.摘要的定义摘要的英文术语:有两个词汇,一个是abstract, 一个是summary.根据美国国家标准学会(American National Standard Institute)于1971年通过并颁布的《美国国家文摘写作标准》(American National Standard for Writing Abstracts)规定,Abstract 不应与summary 混同。
Abstract 对一篇论文的主要内容以精炼的文字进行高度概括,使读者不必阅读全文即可了解论文内容,或者让读者对即将阅读的文章有思想准备,或者让读者判断是否有通读全文的必要。
文中只对论文信息进行浓缩,而不加主观评论或解释,可以脱离原文而独立成篇。
字数通常在100~150个词左右,更确切地说,约为原文长度的1% ~ 5%(有的杂志规定摘要平均为全文的3% ~ 5%)。
现在越来越多的用法是abstract. 尤其是放在索引资料中一律要用abstract 这个术语,在论文的题目下也通常要用这个词。
Summary (概要) 与abstract 无明显差别。
严格地说,summary 一般附在论文的后面,对论文的主要结论和成果进行再叙述。
除臭作用研究(翻译)
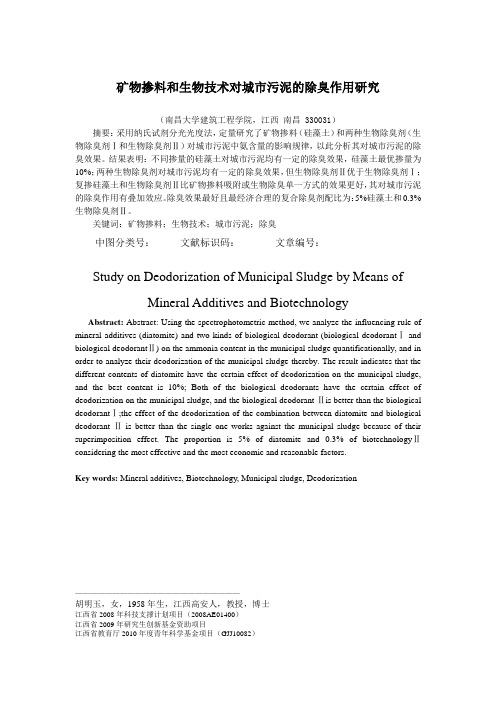
矿物掺料和生物技术对城市污泥的除臭作用研究(南昌大学建筑工程学院,江西南昌 330031)摘要:采用纳氏试剂分光光度法,定量研究了矿物掺料(硅藻土)和两种生物除臭剂(生物除臭剂Ⅰ和生物除臭剂Ⅱ)对城市污泥中氨含量的影响规律,以此分析其对城市污泥的除臭效果。
结果表明:不同掺量的硅藻土对城市污泥均有一定的除臭效果,硅藻土最优掺量为10%;两种生物除臭剂对城市污泥均有一定的除臭效果,但生物除臭剂Ⅱ优于生物除臭剂Ⅰ;复掺硅藻土和生物除臭剂Ⅱ比矿物掺料吸附或生物除臭单一方式的效果更好,其对城市污泥的除臭作用有叠加效应。
除臭效果最好且最经济合理的复合除臭剂配比为:5%硅藻土和0.3%生物除臭剂Ⅱ。
关键词:矿物掺料;生物技术;城市污泥;除臭中图分类号:文献标识码:文章编号:Study on Deodorization of Municipal Sludge by Means ofMineral Additives and BiotechnologyAbstract: Abstract: Using the spectrophotometric method, we analyze the influencing rule of mineral additives (diatomite) and two kinds of biological deodorant (biological deodorantⅠand biological deodorantⅡ) on the ammonia content in the municipal sludge quantificationally, and in order to analyze their deodorization of the municipal sludge thereby. The result indicates that the different contents of diatomite have the certain effect of deodorization on the municipal sludge, and the best content is 10%; Both of the biological deodorants have the certain effect of deodorization on the municipal sludge, and the biological deodorant Ⅱis better than the biological deodorantⅠ;the effect of the deodorization of the combination between diatomite and biological deodorant Ⅱis better than the single one works against the municipal sludge because of their superimposition effect. The proportion is 5% of diatomite and 0.3% of biotechnologyⅡconsidering the most effective and the most economic and reasonable factors.Key words: Mineral additives, Biotechnology, Municipal sludge, Deodorization——————————————————————胡明玉,女,1958年生,江西高安人,教授,博士江西省2008年科技支撑计划项目(2008AE01400)江西省2009年研究生创新基金资助项目江西省教育厅2010年度青年科学基金项目(GJJ10082)引言Introduction随着我国城市化速度加快,污水处理厂所产生的城市污泥量日益增加。
环境保护前沿—干湿交替变化对土壤中磷形态影响及环境意义
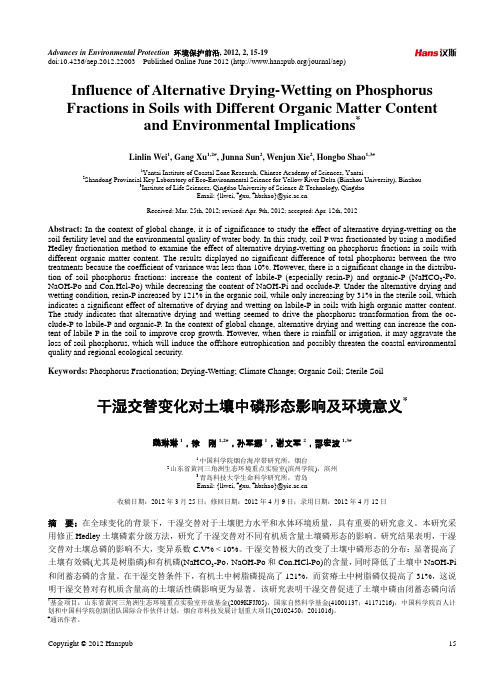
Advances in Environmental Protection 环境保护前沿, 2012, 2, 15-19doi:10.4236/aep.2012.22003 Published Online June 2012 (/journal/aep)Influence of Alternative Drying-Wetting on PhosphorusFractions in Soils with Different Organic Matter Contentand Environmental Implications*Linlin Wei1, Gang Xu1,2#, Junna Sun2, Wenjun Xie2, Hongbo Shao1,3#1Yantai Institute of Coastal Zone Research, Chinese Academy of Sciences, Yantai2Shandong Provincial Key Laboratory of Eco-Environmental Science for Yellow River Delta (Binzhou University), Binzhou3Institute of Life Sciences, Qingdao University of Science & Technology, QingdaoEmail: {llwei, #gxu, #hbshao}@Received: Mar. 25th, 2012; revised: Apr. 9th, 2012; accepted: Apr. 12th, 2012Abstract: In the context of global change, it is of significance to study the effect of alternative drying-wetting on the soil fertility level and the environmental quality of water body. In this study, soil P was fractionated by using a modified Hedley fractionation method to examine the effect of alternative drying-wetting on phosphorus fractions in soils with different organic matter content. The results displayed no significant difference of total phosphorus between the two treatments because the coefficient of variance was less than 10%. However, there is a significant change in the distribu-tion of soil phosphorus fractions: increase the content of labile-P (especially resin-P) and organic-P (NaHCO3-Po, NaOH-Po and Con.Hcl-Po) while decreasing the content of NaOH-Pi and occlude-P. Under the alternative drying and wetting condition, resin-P increased by 121% in the organic soil, while only increasing by 31% in the sterile soil, which indicates a significant effect of alternative of drying and wetting on labile-P in soils with high organic matter content. The study indicates that alternative drying and wetting seemed to drive the phosphorus transformation from the oc-clude-P to labile-P and organic-P. In the context of global change, alternative drying and wetting can increase the con-tent of labile P in the soil to improve crop growth. However, when there is rainfall or irrigation, it may aggravate the loss of soil phosphorus, which will induce the offshore eutrophication and possibly threaten the coastal environmental quality and regional ecological security.Keywords: Phosphorus Fractionation; Drying-Wetting; Climate Change; Organic Soil; Sterile Soil干湿交替变化对土壤中磷形态影响及环境意义*魏琳琳1,徐刚1,2#,孙军娜1,谢文军2,邵宏波1,3#1中国科学院烟台海岸带研究所,烟台2山东省黄河三角洲生态环境重点实验室(滨州学院),滨州3青岛科技大学生命科学研究所,青岛Email: {llwei, #gxu, #hbshao}@收稿日期:2012年3月25日;修回日期:2012年4月9日;录用日期:2012年4月12日摘要:在全球变化的背景下,干湿交替对于土壤肥力水平和水体环境质量,具有重要的研究意义。
容器具清洗SOP中英文
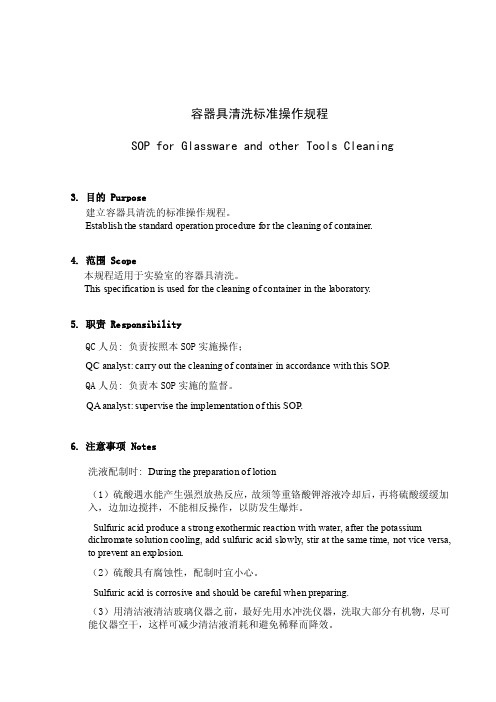
容器具清洗标准操作规程SOP for Glassware and other Tools Cleaning3. 目的 Purpose建立容器具清洗的标准操作规程。
Establish the standard operation procedure for the cleaning of container.4. 范围 Scope本规程适用于实验室的容器具清洗。
This specification is used for the cleaning of container in the laboratory.5. 职责 ResponsibilityQC人员: 负责按照本SOP实施操作;QC analyst: carry out the cleaning of container in accordance with this SOP.QA人员: 负责本SOP实施的监督。
QA analyst: supervise the implementation of this SOP.6. 注意事项 Notes洗液配制时: During the preparation of lotion(1)硫酸遇水能产生强烈放热反应,故须等重铬酸钾溶液冷却后,再将硫酸缓缓加入,边加边搅拌,不能相反操作,以防发生爆炸。
Sulfuric acid produce a strong exothermic reaction with water, after the potassiumdichromate solution cooling, add sulfuric acid slowly, stir at the same time, not vice versa, to prevent an explosion.(2)硫酸具有腐蚀性,配制时宜小心。
Sulfuric acid is corrosive and should be careful when preparing.(3)用清洁液清洁玻璃仪器之前,最好先用水冲洗仪器,洗取大部分有机物,尽可能仪器空干,这样可减少清洁液消耗和避免稀释而降效。
基于连续小波变换的土壤有机质含量高光谱反演
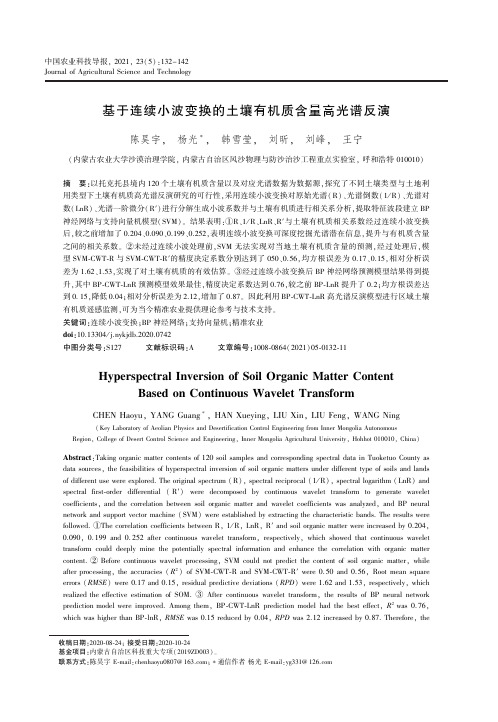
中国农业科技导报ꎬ2021ꎬ23(5):132-142JournalofAgriculturalScienceandTechnology㊀收稿日期:2020 ̄08 ̄24ꎻ接受日期:2020 ̄10 ̄24㊀基金项目:内蒙古自治区科技重大专项(2019ZD003)ꎮ㊀联系方式:陈昊宇E ̄mail:chenhaoyu0807@163.comꎻ∗通信作者杨光E ̄mail:yg331@126.com基于连续小波变换的土壤有机质含量高光谱反演陈昊宇ꎬ㊀杨光∗ꎬ㊀韩雪莹ꎬ㊀刘昕ꎬ㊀刘峰ꎬ㊀王宁(内蒙古农业大学沙漠治理学院ꎬ内蒙古自治区风沙物理与防沙治沙工程重点实验室ꎬ呼和浩特010010)摘㊀要:以托克托县境内120个土壤有机质含量以及对应光谱数据为数据源ꎬ探究了不同土壤类型与土地利用类型下土壤有机质高光谱反演研究的可行性ꎬ采用连续小波变换对原始光谱(R)㊁光谱倒数(1/R)㊁光谱对数(LnR)㊁光谱一阶微分(Rᶄ)进行分解生成小波系数并与土壤有机质进行相关系分析ꎬ提取特征波段建立BP神经网络与支持向量机模型(SVM)ꎮ结果表明:①R㊁1/R㊁LnR㊁Rᶄ与土壤有机质相关系数经过连续小波变换后ꎬ较之前增加了0.204㊁0.090㊁0.199㊁0.252ꎬ表明连续小波变换可深度挖掘光谱潜在信息ꎬ提升与有机质含量之间的相关系数ꎮ②未经过连续小波处理前ꎬSVM无法实现对当地土壤有机质含量的预测ꎬ经过处理后ꎬ模型SVM ̄CWT ̄R与SVM ̄CWT ̄Rᶄ的精度决定系数分别达到了050㊁0.56ꎬ均方根误差为0.17㊁0.15ꎬ相对分析误差为1.62㊁1.53ꎬ实现了对土壤有机质的有效估算ꎮ③经过连续小波变换后BP神经网络预测模型结果得到提升ꎬ其中BP ̄CWT ̄LnR预测模型效果最佳ꎬ精度决定系数达到0.76ꎬ较之前BP ̄LnR提升了0.2ꎻ均方根误差达到0 15ꎬ降低0.04ꎻ相对分析误差为2.12ꎬ增加了0.87ꎮ因此利用BP ̄CWT ̄LnR高光谱反演模型进行区域土壤有机质遥感监测ꎬ可为当今精准农业提供理论参考与技术支持ꎮ关键词:连续小波变换ꎻBP神经网络ꎻ支持向量机ꎻ精准农业doi:10.13304/j.nykjdb.2020.0742中图分类号:S127㊀㊀㊀文献标识码:A㊀㊀㊀文章编号:1008 ̄0864(2021)05 ̄0132 ̄11HyperspectralInversionofSoilOrganicMatterContentBasedonContinuousWaveletTransformCHENHaoyuꎬYANGGuang∗ꎬHANXueyingꎬLIUXinꎬLIUFengꎬWANGNing(KeyLaboratoryofAeolianPhysicsandDesertificationControlEngineeringfromInnerMongoliaAutonomousRegionꎬCollegeofDesertControlScienceandEngineeringꎬInnerMongoliaAgriculturalUniversityꎬHohhot010010ꎬChina)Abstract:Takingorganicmattercontentsof120soilsamplesandcorrespondingspectraldatainTuoketuoCountyasdatasourcesꎬthefeasibilitiesofhyperspectralinversionofsoilorganicmattersunderdifferenttypeofsoilsandlandsofdifferentusewereexplored.Theoriginalspectrum(R)ꎬspectralreciprocal(1/R)ꎬspectrallogarithm(LnR)andspectralfirst ̄orderdifferential(Rᶄ)weredecomposedbycontinuouswavelettransformtogeneratewaveletcoefficientsꎬandthecorrelationbetweensoilorganicmatterandwaveletcoefficientswasanalyzedꎬandBPneuralnetworkandsupportvectormachine(SVM)wereestablishedbyextractingthecharacteristicbands.Theresultswerefollowed.①ThecorrelationcoefficientsbetweenRꎬ1/RꎬLnRꎬRᶄandsoilorganicmatterwereincreasedby0.204ꎬ0.090ꎬ0.199and0.252aftercontinuouswavelettransformꎬrespectivelyꎬwhichshowedthatcontinuouswavelettransformcoulddeeplyminethepotentiallyspectralinformationandenhancethecorrelationwithorganicmattercontent.②BeforecontinuouswaveletprocessingꎬSVMcouldnotpredictthecontentofsoilorganicmatterꎬwhileafterprocessingꎬtheaccuracies(R2)ofSVM ̄CWT ̄RandSVM ̄CWT ̄Rᶄwere0.50and0.56ꎬRootmeansquareerrors(RMSE)were0.17and0.15ꎬresidualpredictivedeviations(RPD)were1.62and1.53ꎬrespectivelyꎬwhichrealizedtheeffectiveestimationofSOM.③AftercontinuouswavelettransformꎬtheresultsofBPneuralnetworkpredictionmodelwereimproved.AmongthemꎬBP ̄CWT ̄LnRpredictionmodelhadthebesteffectꎬR2was0.76ꎬwhichwashigherthanBP ̄lnRꎬRMSEwas0.15reducedby0.04ꎬRPDwas2.12increasedby0.87.ThereforeꎬtheBP ̄CWT ̄LnRhyperspectralinversionmodelcouldprovidetheoreticalreferenceandtechnicalsupportforprecisionagriculture.Keywords:continuouswavelettransformꎻBPneuralnetworkꎻsupportvectormachineꎻprecisionagriculture㊀㊀精准农业作为目前农业发展的主要方向ꎬ是一种基于信息和知识管理的现代化生产系统ꎬ主要是通过3S(GPS㊁GIS和RS)技术与现代农业相结合ꎬ最大限度地提高农业生产力ꎮ所以快速㊁无损㊁精确地获取土壤中水分㊁养分的空间分布成为了实现精准农业的关键环节ꎬ近年来ꎬ光谱分析在土壤化学分析领域得到了迅猛发展ꎬ为实现土壤养分的快速诊断提供了新思路[1]ꎮ有机质是土壤养分供应能力和肥力的重要指标之一ꎬ在全球碳循环中发挥着重要作用ꎮ因此ꎬ快速准确地估测土壤有机质含量对于发展精准农业具有重要意义[2]ꎮ传统的土壤有机质测定方法虽然精度比较高ꎬ但周期较长㊁成本较高ꎬ只能达到瞬测量ꎬ很难进行长时间大面积测量ꎮ高光谱遥感具有波段多㊁波段窄㊁信息丰富和实时高效等特点ꎬ为快速测量土壤有机质含量提供了一种新的方法和手段[3]ꎮ国内外已经有大量研究表明ꎬ通过对光谱数据进行不同的数学变换(主要通过对光谱进行倒数㊁对数㊁微分㊁平方根㊁吸收峰深度㊁包络线去除等方法)可以有效提高光谱数据与土壤有机质含量之间的相关系数ꎬ有效筛选出光谱信息中的敏感波段[4]ꎮ现在各学者主要将研究重心放到了模型建立上[5]ꎬ普遍运用的线性模型有多元逐步回归与偏最小二乘回归[6]ꎻ常见的非线性模型包括BP神经网络[7]㊁支持向量机[8]㊁决策树[9]等ꎬ而且随着非线性模型算法的逐步改良与完善ꎬ在土壤有机质含量估算中已经成为不可取代的一部分ꎮ随着小波算法的改进与发展ꎬ最初仅运用于植物叶绿素㊁冠层成分含量预测中[10 ̄11]ꎬ目前已成为土壤养分预测的热点问题[12 ̄13]ꎬ连续小波变换是目前被广泛应用的一种方法ꎮ王祥浩[14]选择土地裸露地区为样区ꎬ利用神经网络算法对光谱连续小波变换㊁一阶导数㊁对光谱的平均值处理㊁光谱背景及深度4种方法建模ꎬ模型结果表明ꎬ小波变换方法得到的神经网络模型精度最高ꎻ包青岭等[15]选择渭干河-库车河三角洲具有代表性的干旱区绿洲为研究区ꎬ对光谱进行8层分解ꎬ结果表明小波变换不同分解层ꎬ从低频到高频范围内与土壤有机质含量的相关性呈现先减后增的趋势ꎬ结合随机森岭模型可以对干旱区土壤有机质含量进行有效的估算ꎻ王延仓等[16]以北京东部区潮土为例ꎬ对不同梯度重采样的光谱进行连续小波变换后ꎬ利用偏最小二乘法建立模型ꎬ结果表明连续小波分析算法可深入挖掘土壤光谱内的有益信息ꎬ提升对有机质含量的估测能力ꎬ与土壤高光谱反射率相比ꎬ经连续小波技术处理后ꎬ模型精度得到了有效的提升ꎻ叶红云等[17]同样针对干旱区土壤ꎬ通过对两种常用光谱变换Rᶄ㊁Ln(1/R)进行连续小波变换建立偏最小二乘模型ꎬ结果表明连续小波变换不会因人类干扰程度的提高而使模型精度大幅度降低ꎬ更加适用于干旱区有机质含量的预测ꎻ林鹏达等[18]通过解决黑土有机质高光谱野外反演的困难ꎬ同样证明了连续小波变换可有效提升模型精度ꎮ小波技术在土壤有机质高光谱反演研究中逐渐趋于成熟ꎬ但目前学者的研究多数都在同一土壤类型下或同一区域内ꎬ对于不同土壤类型及土地利用下土壤有机质高光谱反演是否存在影响的研究目前并不多ꎮ本文研究区内土壤类型主要包括3类:沙壤土㊁栗钙土㊁盐碱土ꎬ且部分区域土壤盐渍化程度严重ꎬ导致土壤养分空间分布上存在较大差异ꎬ取样表层土地利用类型主要包括:耕地㊁林地㊁草地㊁盐渍地㊁荒地ꎮ通过对原始光谱(R)㊁原始光谱倒数(1/R)㊁原始光谱对数(LnR)以及原始光谱一阶微分(Rᶄ)4种不同情况进行连续小波变换ꎬ利用BP神经网络以及支持向量机2种模型ꎬ探究了不同土壤类型与不同土地利用类型下是否会对土壤有机质高光谱反演模型产生影响ꎬ小波变换前后土壤有机质反演模型的精度ꎬ旨为区域土壤有机质含量监测及实现精准农业提供理论与技术支持ꎮ1㊀材料与方法1.1㊀研究区概况托克托县隶属于内蒙古自治区呼和浩特市ꎬ位于自治区中部㊁大青山南麓㊁黄河上中游分界处北岸的土默川平原上(图1)ꎮ地理坐标东经3315期陈昊宇等:基于连续小波变换的土壤有机质含量高光谱反演111ʎ2ᶄ30ᵡ 111ʎ32ᶄ21ᵡ㊁北纬40ʎ5ᶄ55ᵡ 40ʎ35ᶄ15ᵡꎬ总面积1409.67km2ꎬ平均海拔1117mꎬ属于温带大陆性干旱气候ꎬ年均气温7.3ħꎬ年均降雨362mmꎮ托克托县耕地总面积达400km2ꎬ其中古城镇㊁新营子镇和五申镇的耕地较多ꎬ占全县耕地面积的60%以上[19]ꎬ主要作物包括小麦㊁玉米㊁莜麦ꎮ工农业及生产生活用水主要来源于大黑河和黄河水资源ꎬ整个地形以大黑河为轴ꎬ呈现由丘陵向平原过渡的趋势ꎬ地势为东南高㊁西北和西南低ꎮ东南向西北土壤类型依次为栗钙土㊁砂壤石灰性冲积土㊁盐渍化石灰性冲积土[20]ꎬ土壤类型的不同导致土壤养分存在差异性分布ꎮ植被类型从西向东依次为草甸草原㊁干草原和退化灌丛草原分布ꎮ以Landsat8OLI影像为基础数据源ꎬ运用人工目视解译与BP神经网络分类法得到托克托县2019年7月份土地利用数据ꎬ其中耕地面积最大为730.12km2ꎬ占51.79%ꎻ林草地338.7km2ꎬ占24.02%ꎻ盐碱地141.1km2ꎬ占10.00%ꎮ详细土地利用空间分布见图1ꎮ图1㊀土样采集点及土地利用空间分布Fig.1㊀Collectionpointsofsoilsamplesandspatialdistributionoflanduse1.2㊀研究方法1.2.1㊀土样采集与处理㊀土壤样本点均匀地分布在托克托县境内ꎬ采集方法为五点采样法ꎬ采集深度为0 20cmꎬ共采集120个点ꎮ采集的土样置于通风干燥室内进行自然风干㊁研磨ꎬ过10目筛ꎬ进行土壤光谱测定ꎻ过100目筛ꎬ采用重铬酸钾外加热法进行土壤有机质含量测定ꎮ1.2.2㊀光谱测量及光谱处理㊀土壤光谱于暗室内测量ꎬ采用SVCHR ̄1024(北京东方佳气科技有限公司)便携式光谱仪ꎬ光谱范围在350~2500nmꎮ在350~1000nm波段之间光谱分辨率ɤ3.5nmꎻ在1000~1850nm波段之间ꎬ光谱分辨率ɤ9.5nmꎻ在1850~2500nm波段之间ꎬ光谱分辨率ɤ6.5nmꎮ光源采用与太阳光接近的50W卤素灯ꎬ将土壤样品放入深2cm㊁宽10cm的黑色器皿内ꎬ用直尺将土壤表面刮平ꎬ探头距离土样10cmꎬ光源距离土壤表面30cmꎬ天顶角为15ʎꎮ测量前用白板进行标定ꎬ每个土样采集5条光谱作为该土样的光谱数据ꎮ由于受噪音与仪器暗电流的的影响ꎬ导致光谱数据混入噪音等信息ꎬ因此删除350~399nm和2400~2500nm的波段ꎬ采用五点平滑法对光谱进行平滑处理ꎬ并将光谱重采样至5nmꎬ同时对原始光谱(R)进行一阶微分(Rᶄ)㊁倒数(1/R)㊁对数(LnR)等传统数学变换ꎮ1.2.3㊀连续小波变换㊀采用连续小波变换ꎬ并用Mexh小波母函数对原始光谱㊁原始光谱的倒数㊁对数㊁一阶微分进行10层小波变换ꎬ生成一系列小波系数ꎮΨaꎬb=1㊀aΨλ-baæèçöø÷(1)式中ꎬa为伸缩因子ꎬb为平移因子ꎬλ为土壤高光谱数据的波段数ꎮWfaꎬb()=fꎬΨaꎬb()=ʏ+ɕ-ɕfλ()Ψaꎬbλ()dy(2)式中ꎬfλ()为土壤光谱反射率ꎬ小波系数Wfaꎬb()包含二维ꎬ分别为波长(350~2500)与分解尺度(1ꎬ2ꎬ3 10)ꎬ故小波系数行为尺度数ꎬ列为波长数的矩阵[16]ꎮ1.2.4㊀模型及精度验证㊀采用BP神经网络与支持向量机模型(supportvectormachineꎬSVM)建立土壤有机质预测模型ꎬ支持向量机采用线性核函数ꎬ相对于径向基函数(radialbasisfunctionꎬ431中国农业科技导报23卷RBF)来说计算高效ꎬ不易过拟合ꎮBP神经网络的迭代次数设置为1000ꎬ学习率0.01ꎬ训练的均方根误差(rootmeansquareerrorꎬRMSE)小于0.001ꎮ依据相关系数筛选的特征波段以及小波系数作为自变量ꎬ土壤有机质含量为因变量ꎬ分别建立模型ꎬ模型精度采用决定系数(R2)㊁均方根误差(RMSE)㊁相对分析误差(relativepercentdeviationꎬRPD)以及1ʒ1线共同评价ꎮR2表征模型的稳定性ꎬ越接近于1模型越稳定ꎬ拟合程度越好ꎮ均方根误差(RMSE)用来检验模型的预报能力ꎬRMSE越小则表明模型的估测能力越好ꎮRPD是样本的标准差与RMSE的比值ꎬRPD<1.4时ꎬ模型无法对样品进行预测ꎻ1.4ɤRPD<2时ꎬ模型效果一般ꎬ可以用来对样品进行粗略评估ꎻRPDȡ2时ꎬ模型具有极好的预测能力ꎮ1ʒ1线表示实测值与预测值构成的点偏离y=x线的程度[21]ꎮ2㊀结果与分析2.1㊀土壤有机质含量统计分析建模样品集㊁不同土地利用方式㊁不同土壤类型下土壤有机质含量描述性统计见表1ꎮ本研采样点内土地利用方式主要包括林地㊁草地㊁耕地㊁盐渍地ꎬ土壤有机质在草地内均值含量最大(0 80%)ꎬ其次为林地(0.72%)㊁耕地(0.67%)㊁盐渍地有机质含量最低(0.63%)ꎻ土壤有机质含量最大值位于耕地(1.28%)ꎬ最小值位于林地(0 19%)ꎮ采样点内主要土壤类型为栗钙土㊁沙壤土㊁盐碱土ꎬ沙壤土有机质含量最高(0.77%)ꎬ其次为盐碱土(0.68%)和栗钙土(0.67%)ꎬ土壤有机质含量最大值位于沙壤土内(1.28%)ꎬ最小值位于盐碱土内(0.19%)ꎮ表1㊀土壤有机质含量描述性统计结果Table1㊀Descriptivestatisticsresultsoforganicmattercontentinsoilsamples项目Item样品集及类型Samplesetandtype土样数Numberofsamples最大值Maximum/%最小值Minimumvalue/%均值Meanvalue/%标准差Standarddeviation/%模型样品集Modelsampleset样品全集Wholeset1201.200.1940.710.276建模集Modelingset901.200.190.720.227验证集Validationset301.280.250.70.257土地利用方式Landusepattern耕地Cultivatedland551.280.200.670.20林地Woodland201.150.190.720.23草地Grassland251.180.250.800.21盐渍地Salinesoil201.030.250.630.22土壤类型Soiltype栗钙土Chestnutsoil541.20.20.670.22沙壤土Sandyloam511.280.250.770.24盐碱土Saline ̄alkalisoil151.150.190.680.222.2㊀土壤反射光谱特征对R㊁1/R㊁LnR㊁Rᶄ进行小波变换ꎬ变换结果如图2所示ꎬR㊁1/R㊁LnR光谱曲线较为平滑ꎬ分解曲线随波峰波谷变化.Rᶄ其光谱曲线并不规则存在较多波峰波谷ꎬ分解小波系数与前三者不同ꎮR㊁1/R㊁LnR㊁Rᶄ分解后ꎬ小波系数均随分解尺度的增加而增加ꎬ同时可以看出ꎬ由Mexh小波母函数进行的连续小波变换ꎬ对于光谱波峰与波谷有较高的敏感性ꎬ对于放大㊁挖掘光谱信息有着显著的作用ꎮ2.3㊀相关性分析2.3.1㊀不同导数变换光谱与土壤有机质含量相关性㊀土壤有机质含量与光谱相关性曲线及敏感波段见图3ꎮR与土壤有机质含量呈负相关关系(相关系数r=-0.463)ꎬ主要集中于735~780nm处波段ꎻ1/R与土壤有机质的相关性则与R相反ꎬ呈正相关关系(r=0.462)ꎬ集中于600~800nm与1800~2200nm处波段ꎻLnR的相关性曲线图与R相关性曲线类似ꎬ总体呈现负相关关系ꎬ相关系数(r=-0.465)ꎬ主要集中于745~7955315期陈昊宇等:基于连续小波变换的土壤有机质含量高光谱反演nm处的波段ꎻRᶄ相关性在500nm(r=-0.589)与1400nm(r=-0.411)处为负相关ꎬ在800nm(r=0.408)与1380nm(r=0.412)处为正相关ꎬ相关系数曲线变换趋势与前三者不同ꎬ呈无规律变化ꎮ2.3.2㊀不同分解尺度小波系数与土壤有机质含量的相关性㊀图4为不同光谱变换方式经过连续小波变换后与土壤有机质含量的相关系数矩阵图ꎬ其中红色代表相关性高的区域ꎬ蓝色代表相关性低的区域ꎮR在800~1000㊁1400~1600nm处相关性明显增加ꎬ在500㊁800㊁2200nm波段处相关系数达到最大值(r=0.667)ꎻ1/R在800~1200nm处相关系数达到最大值(r=0.552)ꎬ在2400~2500nm处相系数达到0.4ꎬ受噪音和仪器本身的影响ꎬ此波段的相关系数不进行相关性参考ꎻLnR在分解尺度1下相关性较低ꎬ在2~10尺度下ꎬ相关性出现最大值(r=0.664)ꎻRᶄ相关性主要集中在500~900㊁1200~1600㊁2100~2300nm处ꎮ筛选的敏感波段与尺度如表2所示ꎮ有效的光谱信息主要存在于低分解尺度ꎬ随分解尺度的增加呈递减趋势ꎬ相关性最大值较未处理前分别增加了0.204㊁0.09㊁0.199㊁0.252ꎬ对于挖掘潜在光谱信息有着重要意义ꎮ2.4㊀土壤有机质高光谱模型建立2.4.1㊀BP神经网络预测模型㊀采用BP神经网络构建反演模型ꎬ结果如表3所示ꎮ未进行连续小波变换处理的模型中ꎬBP ̄R与BP ̄Rᶄ效果较好ꎬR2分别为0.69和0.73ꎬRPD为1.45与1.53ꎬ模型能粗略估算土壤有机含量ꎬBP ̄LnR与BP ̄1/R样本外预测能力较差ꎬ同时RPD未达到1.4以上ꎬ不能对土壤有机质未能进行有效预测ꎻ连续小图2㊀连续小波变换光谱特性Fig.2㊀Spectralcharacteristicsofcontinuouswavelettransform631中国农业科技导报23卷图3㊀土壤光谱相关性曲线及敏感波段Fig.3㊀Correlationcurveandsensitivebandofsoilspectrum图4㊀土壤有机质与小波系数相关性Fig.4㊀Correlationbetweensoilorganicmatterandwaveletcoefficients7315期陈昊宇等:基于连续小波变换的土壤有机质含量高光谱反演表2㊀筛选的敏感波段Table2㊀Sensitivebandforscreening处理方式Treatmentmethod相关系数Correlationcoefficient分解尺度Decompositionscale敏感波段Sensitiveband/nmCWT ̄R0.6671~10214㊁212㊁91㊁91㊁109㊁109㊁109㊁110㊁111㊁112CWT ̄1/R0.5521~7400㊁212㊁108㊁108㊁108㊁108㊁109CWT ̄LnR0.6642~8213㊁212㊁45㊁45㊁45㊁109㊁110CWT ̄Rᶄ0.6641~10212㊁215㊁215㊁216㊁99㊁99㊁99㊁98㊁98㊁98波变换处理之后的模型ꎬ仅BP ̄CWT ̄1/R模型RPD未达到预测水平ꎬ其余3种模型R2与RPD较未处理前均有所增加ꎬRMSE均减少ꎬ其中BP ̄CWT ̄LnR模型预测效果较好ꎬRPD达到2.12可以有效地对土壤有机质进行预测ꎮ将BP ̄CWT处理的4个模型的实测值与预测值进行1ʒ1线分析ꎮ由图5可知ꎬ除BP ̄CWT ̄1/R模型外ꎬ其余模型的实测值与预测值样点基本分布在1ʒ1线附近ꎬBP ̄CWT ̄LnR效果较为明显ꎬ且估算精度高ꎬ可较好地进行土壤有机质含量的估算ꎮ2.4.2㊀支持向量机预测模型㊀SVM构建反演模型ꎬ结果如表4所示ꎮ未经过连续小波处理的光谱特征波段未能较好地对土壤有机质进行预测反演ꎬ经过CWT后模型SVM ̄CWT ̄R与SVM ̄CWT ̄Rᶄ预测结果较之前有较大的提升ꎬR2分别达到了0.50与0.56ꎬ二者RPD均达到1.4以上ꎬ可以粗表3㊀土壤有机质BP神经网络估测模型结果Table3㊀ResultsofBPneuralnetworkestimationmodelforsoilorganicmatter模型Model建模集ModelingsetR2RMSE验证集ValidationsetR2RMSERPDBP ̄R0.690.170.520.191.45BP ̄1/R0.680.170.330.221.25BP ̄LnR0.560.190.240.221.25BP ̄Rᶄ0.730.160.520.181.53BP ̄CWT ̄R0.800.140.540.171.62BP ̄CWT ̄1/R0.640.180.210.280.98BP ̄CWT ̄LnR0.760.150.740.132.12BP ̄CWT ̄Rᶄ0.770.140.660.161.72表4㊀土壤有机质支持向量机估测模型结果Table4㊀Supportvectormachineestimationmodelresultsofsoilorganicmatter模型Model建模集ModelingsetR2RMSE验证集ValidationsetR2RMSERPDSVM ̄R0.210.200.190.201.38SVM ̄1/R0.210.200.200.211.31SVM ̄LnR0.210.200.200.211.31SVM ̄Rᶄ0.430.170.270.201.38SVM ̄CWT ̄R0.500.160.480.171.62SVM ̄CWT ̄1/R0.290.190.160.211.31SVM ̄CWT ̄LnR0.490.160.270.201.38SVM ̄CWT ̄Rᶄ0.560.150.410.181.53831中国农业科技导报23卷图5㊀BP ̄CWT模型土壤实测值与预测值对比Fig.5㊀ComparisonofmeasuredvalueandpredictedvalueofBP ̄CWTmodel略地对土壤有机质进行预测ꎮ同时根据图6ꎬSVM ̄CWT模型进行1:1线分析ꎬ二者实测值与预测值分布情况在4种模型下较好ꎬ虽然模型SVM ̄CWTLnR分布同样较为集中ꎬ但其样本外预测情况较差(RPD=1.38)ꎬ综合考虑不对其进行土壤有机质预测ꎮ结合表3和表4的结果分析ꎬ连续小波变换能够有效地提升模型精度与模型泛化能力ꎬ对于光谱信息挖掘有着重要意义ꎬBP神经网络与支持向量机对CWT ̄R与CWT ̄Rᶄ都能够提升R2减少RMSEꎬ可对土壤有机质做出较好的预测ꎮ虽然BP神经网络与支持向量机在处理非线性回归问题中有较强的能力ꎬ但本身模型中存在不稳定性ꎬ对模型的环境设置同样要求较高ꎬ所以未能对所有数据集进行良好的预测ꎮ3㊀讨论本研究采用连续小波变换对光谱进行处理ꎬ用BP神经网络与支持向量机(SVM)两种模型对土壤有机质含量进行反演预测ꎮ未经过连续小波变换前ꎬR㊁1/R㊁LnR㊁Rᶄ与土壤有机质的相关系系数最大值分别为-0.463㊁0.462㊁-0.465㊁0.589ꎬ可以看出ꎬRᶄ与土壤有机质的相关系数最高ꎬ与吴倩等[22]㊁张新乐等[23]的研究结果相同ꎻ经过连续小波变换后ꎬCWT ̄R㊁CWT ̄1/R㊁CWT ̄LnR㊁CWT ̄Rᶄ相关系数最大值分别为0 667㊁0.552㊁0 664㊁0.662ꎬ较之前分别增加了0 20㊁0.09㊁0.19㊁0.07ꎮ王延仓等[1]㊁于雷等[4]㊁叶红云等[17]等同样证明连续小波变换可有效提高与土壤有机质含量的相关系数ꎮ不同分解尺度对于光谱数据的深度挖掘有着重要意义ꎬ本研究只利用Mexh小波母函数进行处理ꎬ未对其他函数进行考虑ꎬ分解层数同样是根据前人经验所得[4ꎬ10]ꎬ小波技术的研究与发展仍然有很大的探索空间ꎮ相对于两种模型来看ꎬ未进行连续小波处理9315期陈昊宇等:基于连续小波变换的土壤有机质含量高光谱反演图6㊀利用SVM ̄CWT模型土壤实测值与预测值的对比Fig.6㊀ComparisonofsoilmeasuredvalueandpredictedvalueofSVM ̄CWTmodel的支持向量机模型中ꎬ只有SVM ̄Rᶄ模型R2最高达到0.43ꎬ其余三者均未到达0.4ꎮ综合多种模型评价方法ꎬ由于其RPD未达到1.4以上ꎬ无法对土壤有机质含量进行预测ꎮ经过连续小波处理后ꎬ各模型的R2有明显提高ꎬ其中SVM ̄CWT ̄R与SVM ̄CWT ̄Rᶄ模型效果较好ꎬR2分别提高了0.29㊁0.13ꎬRPD达到1.62与1.53实现了对土壤有机质有效的预测ꎬ但预测结果较BP神经网络较低ꎮ在BP神经网络预测模型中ꎬ未进行连续小波变换前ꎬBP ̄R与BP ̄Rᶄ预测效果较好ꎬR2达到0.69与0.73ꎬRPD为1.45与1.53ꎻ进行连续小波处理后ꎬ除SVM ̄CWT ̄1/R模型未到达预测效果ꎬ其余3种模型预测结果较之前均有明显改善ꎬ可实现对土壤有机质较好的预测ꎬ其中BP ̄CWT ̄LnR预测模型效果最佳R2达到0.76ꎬRPD达到2.12ꎮ根据1:1线分析图也可看出ꎬ其实测值与预测值分布较为集中ꎬ于雷等[4]㊁叶红云等[17]㊁林鹏达等[18]同样通过连续小波变换有效提升了模型的精度与泛化能力ꎮ针对土壤有机质高光谱反演研究中ꎬ姚聪[24]对耕层土壤通过BP神经网络与支持向量机模型ꎬ反演精度R2分别为0.42与0.67ꎻ叶红云等[17]采用连续小波变换对干旱区土壤有机质反演ꎬ模型精度R2=0.75㊁EMSE=0.71ꎻ谢文[25]在森林土壤有机质反演研究中ꎬBP神经网络模型R2=0 78㊁EMSE=0.77ꎬ支持向量机模型R2=0.87㊁EMSE=0.76ꎮ本研究对耕地㊁林草地㊁盐碱地㊁栗钙土㊁沙壤土㊁盐渍土等不同土地利用类型与土壤类型进行综合反演ꎬ最佳反演模型为BP ̄CWTLnRꎬR2=0.76㊁EMSE=0.15㊁RPD=2.12ꎬ与前人研究的结果基本相符ꎬ证明通过连续小波变换处理ꎬ不同土壤类型与土地利用类型未对土壤反演模型精度产生影响ꎮ所以采用连续小波变换进行光谱数据挖掘ꎬ采用BP ̄CWT ̄LnR神经网络建041中国农业科技导报23卷立反演模型ꎬ可对不同土地利用于土壤类型条件下土壤有机质高光谱反演提供一定的理论支持与应用价值ꎮ参㊀考㊀文㊀献[1]㊀王延仓ꎬ杨秀峰ꎬ赵起超ꎬ等.二进制小波技术定量反演北方潮土土壤有机质含量[J].光谱学与光谱分析ꎬ2019ꎬ39(9):2855-2861.WANGYCꎬYANGXFꎬZHAOQCꎬetal..Quantitativeinversionofsoilbasedonbinarywavelettransform[J].SpectroscopySpectralAnal.ꎬ2019ꎬ39(9):2855-2861. [2]㊀于雷ꎬ洪永胜ꎬ耿雷ꎬ等.基于偏最小二乘回归的土壤有机质含量高光谱估算[J].农业工程学ꎬ2015ꎬ31(14):103-109.YULꎬHONGYSꎬGENGLꎬetal..Hyperspectralestimationofsoilorganicmattercontentbasedonpartialleastsquaresregression[J].Trans.CSAEꎬ2015ꎬ31(14):103-109. [3]㊀钟浩ꎬ李西灿ꎬ翟浩然ꎬ等.耕层土壤有机质高光谱间接估测模型[J].测绘科学技术学报ꎬ2019ꎬ36(1):74-78ꎬ85.ZHONGHꎬLIXCꎬZhAIHRꎬetal..Hyperspectralindirectestimationmodelofsoilorganicmattercontentinploughlayer[J].J.GeomaticsTechnol.ꎬ2019ꎬ36(1):74-78ꎬ85. [4]㊀于雷ꎬ洪永胜ꎬ周勇ꎬ等.连续小波变换高光谱数据的土壤有机质含量反演模型构建[J].光谱学与光谱分析ꎬ2016ꎬ36(5):1428-1433.YULꎬHONGYSꎬZHOUYꎬetal..Inversionofsoilorganicmattercontentusinghyperspectraldatabasedoncontinuouswavelettransformation[J].SpectroscopySpectralAnal.ꎬ2016ꎬ36(5):1428-1433.[5]㊀STENBERGBꎬVISCARRARRAꎬMOUAZENAMꎬeta1..Visibleandnearinfraredspectroscopyinsoilscience[J].Adv.Agron.ꎬ2010ꎬ107:163-215.[6]㊀聂哲ꎬ李秀芬ꎬ吕家欣ꎬ等.东北典型黑土区表层土壤有机质含量高光谱反演研究[J].土壤通报ꎬ2019ꎬ50(6):1285-1293.NIEZꎬLIUXFꎬLYUJXꎬetal..HyperspectralretrievalofsurfacesoilorganicmattercontentinatypicalblacksoilregionofnortheastChina[J].Chin.J.SoilSci.ꎬ2019ꎬ50(6):1285-1293.[7]㊀沈润平ꎬ丁国香ꎬ魏国栓ꎬ等.基于人工神经网络的土壤有机质含量高光谱反演[J].土壤学报ꎬ2009ꎬ46(3):391-397.SHENRPꎬDINGGXꎬWEIGSꎬetal..Retrievalofsoilorganicmattercontentfromhyper ̄spectrumbaseonAnn[J].ActaPedol.Sin.ꎬ2009ꎬ46(3):391-397.[8]㊀沈强ꎬ张世文ꎬ夏沙沙ꎬ等.基于支持向量机的土壤有机质高光谱反演[J].安徽理工大学学报(自然科学版)ꎬ2019ꎬ39(4):39-45.SHENQꎬZHANGSWꎬXIASSꎬetal..Hyperspectralinversionofsoilorganicmatterbasedonsupportvectormachine[J].J.AnhuiUniv.Sci.Technol.(Nat.Sci.)ꎬ2019ꎬ39(4):39-45.[9]㊀BREIMANLꎬFRIEDMANJHꎬOLSHENRAꎬetal..ClassificationandRegressionTrees[M].Belmont:WadsworthInternationalGroupꎬ1984.[10]㊀方圣辉ꎬ乐源ꎬ梁琦.基于连续小波分析的混合植被叶绿素反演[J].武汉大学学报ꎬ2015ꎬ40(3):296-302.FANGSHꎬLEYꎬLIANGQ.Retrievalofchlorophyllcontentusingcontinuouswaveletanalysisacrossarangeofvegetationspecies[J].GeomaticsInform.Sci.WuHanUniv.ꎬ2015ꎬ40(3):296-302.[11]㊀何汝艳ꎬ乔小军ꎬ蒋金豹ꎬ等.小波法反演条锈病胁迫下冬小麦冠层叶片全氮含量[J].农业工程学报ꎬ2015ꎬ31(2):141-146.HERYꎬQIAOXJꎬJIANGJBꎬetal..Retrievingcanopyleaftotalnitrogencontentofwinterwheatbycontinuouswavelettransform[J].Trans.CSAEꎬ2015ꎬ31(2):141-146. [12]㊀陈红艳ꎬ赵庚星ꎬ李希灿ꎬ等.小波分析用于土壤速效钾含量高光谱估测研究[J].中国农业科学ꎬ2012ꎬ45(7):1425-1431.CHENHYꎬZHAOGXꎬLIXCꎬetal..Applicationofwaveletanalysisforestimationofsoilavailablepotassiumcontentwithhyperspectralreflectance[J].Sci.Agric.Sin.ꎬ2012ꎬ45(7):1425-1431.[13]㊀高洪智ꎬ卢启鹏.土壤主要养分近红外光谱分析及其测量系统[J].光谱学与光谱分析ꎬ2011ꎬ31(5):1245-1249.GAOHZꎬLUQP.Nearinfraredspectralanalysisandmeasuringsystemforprimarynutrientofsoil[J].SpectroscopySpectralAnal.ꎬ2011ꎬ31(5):1245-1249.[14]㊀王祥浩.土壤有机质高光谱反演模型研究[J].黑龙江工程学院学报ꎬ2019ꎬ33(5):34-39.WANGXH.Researchonhighspectralinversionmodelofsoilorganicmatter[J].J.HeilongjiangInstituteTechnol.ꎬ2019ꎬ33(5):34-39.[15]㊀包青岭ꎬ丁建丽ꎬ王敬哲ꎬ等.基于随机森林算法的土壤有机质含量高光谱检测[J].干旱区地理ꎬ2019ꎬ42(6):1404-1414.BAOQLꎬDINGJLꎬWANGJZꎬetal..Hyperspectraldetectionoforganicmattercontentbasedonrandomforestalgorithm[J].AridLandGeographyꎬ2019ꎬ42(6):1404-1414.[16]㊀王延仓ꎬ张兰ꎬ王欢ꎬ等.连续小波变换定量反演土壤有机质含量[J].光谱学与光谱分析ꎬ2018ꎬ38(11):3521-3527.WANGYCꎬZHANGLꎬWANGHꎬetal..Quantitativeinversionofsoilorganicmattercontentbasedoncontinuouswavelettransform[J].SpectroscopySpectralAnal.ꎬ2018ꎬ38(11):3521-3527.[17]㊀叶红云ꎬ熊黑钢ꎬ张芳ꎬ等.基于CWT的人类不同程度干扰下干旱区土壤有机质含量估算研究[J].激光与光电子学进展ꎬ2019ꎬ56(5):115-124.YEHYꎬXIONGHGꎬZHANGFꎬetal..CWT ̄Basedestimationofsoilorganicmattercontentinaridareaunderdifferenthumandisturbancedegrees[J].LaserOptoelectronicsProgr.ꎬ2019ꎬ56(5):115-124.[18]㊀林鹏达ꎬ佟志军ꎬ张继权ꎬ等.基于CWT的黑土有机质含量野外高光谱反演模型[J].水土保持研究ꎬ2018ꎬ25(2):46-52ꎬ57.LINPDꎬTONGZJꎬZHANGJQꎬetal..Inversionofblacksoilorganicmattercontentwithfieldhyperspectralreflectancebasedoncontinuouswavelettransformation[J].Res.SoilWaterConnserv.ꎬ2018ꎬ25(2):46-52ꎬ57.1415期陈昊宇等:基于连续小波变换的土壤有机质含量高光谱反演[19]㊀范晓冰ꎬ鲁丽波ꎬ范海娇.托克托县耕地动态变化及驱动因子分析[J].内蒙古师范大学学报ꎬ2017ꎬ46(1):151-155.FANGXBꎬLULBꎬFANGHJꎬetal..AnanalysisofdynamicchangesanddrivingfactorsofarablelandinTogtohcounty[J].J.InnerMongoliaNorm.Univ.ꎬ2017ꎬ46(1):151-155. [20]㊀李政葵.内蒙古托克托县潜水与土壤中氟化物的分布规律及其相关性研究[D].呼和浩特:内蒙古大学ꎬ硕士学位论文ꎬ2015.LIKZ.DistributionandcorrelationoffluoridebetweenunconfinedwaterandsoilofInnerMongoliaTuoketuocounty[D].Hohhot:InnerMongoliaUniversityꎬMasterDissertationꎬ2015.[21]㊀VISCARRARAꎬMCGLYNNRNꎬMCBRATNEYAB.Determiningthecompositionofmineral ̄organicmixesusingUV ̄vis ̄NIRdiffusereflectancespectroscopy[J].Geodermaꎬ2007ꎬ137(1/2):70-82.[22]㊀吴倩ꎬ姜琦刚ꎬ史鹏飞ꎬ等.基于高光谱的土壤碳酸钙含量估算模型研究[J/OL].国土资源遥感ꎬ2020:[2020-09-16].https://kns.cnki.net/kcms/detail/11.2514.P.20200810.1518.004.html.WUQꎬJIANGQGꎬSHIPFꎬetal..Estimationofsoilcalciumcarbonatecontentbasedonhyperspectraldata[J/OL].RemoteSensingLandResourcesꎬ2020:[2020-09-16].https://kns.cnki.net/kcms/detail/11.2514.P.20200810.1518.004.html.[23]㊀张新乐ꎬ于滋洋ꎬ李厚萱ꎬ等.东北水稻叶片SPAD遥感光谱估算模型[J].中国农业大学学报ꎬ2020ꎬ25(1):66-75.ZHANGXLꎬYUZYꎬLIHXꎬetal..RemotesensingestimationmodelofSPADforriceleavesinNortheastChina[J].J.Chin.Agric.Univ.ꎬ2020ꎬ25(1):66-75.[24]㊀姚聪.基于卷积神经网络的耕层土壤有机质含量估测模型研究[D].山东泰安:山东农业大学ꎬ硕士学位论文ꎬ2020.YAOC.Studyonestmationmodelofsoilorganicmattercontentinplowedlayerbasedonconvolutionalneuralnetwork[D].ShandongTaian:ShandongAgriculturalUniversityꎬMasterDissertationꎬ2020.[25]㊀谢文.基于高光谱技术的森林土壤不同养分含量光谱特征及估测模型研究[D].南昌:江西农业大学ꎬ博士学文论文ꎬ2017.XIEW.Studyonspectralcharacteristicsandestimationmodelsofdifferentnutrientcontentsinforestsoilsbasedonhyperspectraltechnology[D].Nanchang:JiangsuAgriculturalUniversityꎬDoctorDissertationꎬ2017.(责任编辑:陈凌云)241中国农业科技导报23卷。
砂岩型铀矿床的有机地球化学分带性及其与铀成矿的关系--以内蒙古皂火壕铀矿床为例

砂岩型铀矿床的有机地球化学分带性及其与铀成矿的关系--以内蒙古皂火壕铀矿床为例孙晔【摘要】内蒙古皂火壕铀矿床是古层间氧化带砂岩型铀矿床,具有明显的有机地球化学分带性。
笔者认为,其过渡带有机质含量高是原地同沉积富集和异地来源有机质“叠加”的结果,二者分别受控于岩相古地理条件和古层间氧化作用中的铀有机质作用。
过渡带中的有机质主要包括腐殖质、有机黏土复合体以及干酪根。
铀对有机质具有热演化催化作用,可能导致了过渡带中的有机质成熟度增高。
皂火壕铀矿床是成岩作用发展到一定阶段的产物,侏罗系煤系地层在成岩演化中产生了丰富的可溶性有机质和烃类物质,它们参与了铀成矿的全过程。
有机地球化学分带性指标可以作为层间氧化带过渡带乃至前锋线定位识别的微观及宏观标志,对铀矿找矿勘探具有重要的指导作用。
%Zaohuohao uranium deposit in Inner Mongolia is of sandstone‐type formed by the paleo‐inter‐layer oxidation. Organic indicators shew obvious geochemical zones which can be used as location mark of transitional zone. It was considered that the high content of organic matters was caused by the superposi‐tion of the sedimentation of organic matters within and outside the transitional zones , which were con‐trolled by lithofacies paleogeography and uranium‐organism reactio n in the paleo interlayer zone respec‐anic matters in the transitional zone contains humus , organic clay complex and kero‐gen. Uranium act as the catalyst to thermal evolution of organic matters. This may cause the high matu‐ration of organic ma tters in the transitional zone.Zaohuohao Uranium Deposit was the product of the dia‐geneticevolution. Organic matters of Jurassic coal measure stratum produced rich soluble organic matter and hydrocarbons which participated in all the process of uranium mineralization. This study broadened the research field of organic geologic process of sandstone type uranium deposit .【期刊名称】《铀矿地质》【年(卷),期】2016(032)003【总页数】8页(P129-136)【关键词】砂岩型铀矿;煤系有机质;有机地球化学分带;有机质的成岩作用【作者】孙晔【作者单位】中核集团地矿事业部/中国核工业地质局,北京 100013【正文语种】中文【中图分类】P593我国北方沉积盆地已发现并探明了一批大型、特大型砂岩型铀矿床。
GLP(良好实验室规范)
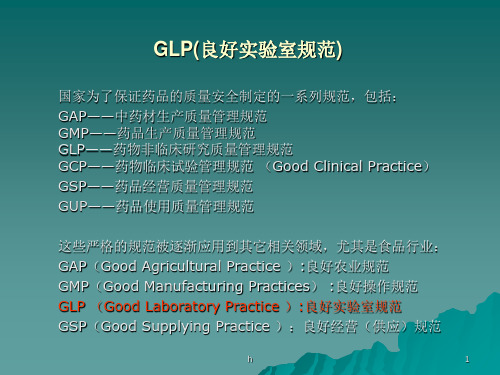
2、安全规范
SAFETY PRACTICE
3、符合专业要求的操作
GOOD OPERATIONAL PRACTICE
4、纯净水
PURIFIED WATER
5、标准物质
REFERENCE MATERIALS
6、标准溶液
STANDARD SOLUTIONS
7、留样
RETAINED SAMPLES
8、实验室器皿的清洁
Cylinders shall be fixed and clearly labeled.
钢瓶应清楚标识,并固定。
Cuts and grazes to the skin are adequately covered by suitable dressings.
伤口和皮肤擦破处应适当地包扎敷裹。
Personnel will not work in a laboratory alone.
CLEANING OF LABORATORY WARE
9、化学通风橱
FUME CUPBOARDS
10、层流通风柜(超净工作台) LAMINAR FLOW HOODS
11、实验室能力验证
LABORATORY PROFICIENCY TEST
h
4
GLP(良好实验室规范)
1、GOOD HOUSEKEEIPNG PRACTICE 整洁有序的实验室日常管理
应穿着紧身的棉质工作服,工作服不可穿进茶点休息区域或食堂。
Eye protection will be worn unless the analyst can demonstrate that adequate protection is provided by other means.
Stabilization mechanisms of soil organic matter- Implications for C-saturation of soils
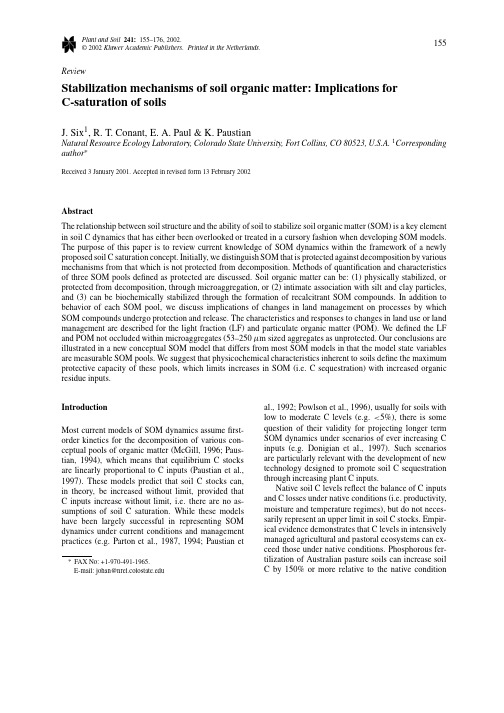
Plant and Soil241:155–176,2002.©2002Kluwer Academic Publishers.Printed in the Netherlands.155 ReviewStabilization mechanisms of soil organic matter:Implications forC-saturation of soilsJ.Six1,R.T.Conant,E.A.Paul&K.PaustianNatural Resource Ecology Laboratory,Colorado State University,Fort Collins,CO80523,U.S.A.1Corresponding author∗Received3January2001.Accepted in revised form13February2002AbstractThe relationship between soil structure and the ability of soil to stabilize soil organic matter(SOM)is a key element in soil C dynamics that has either been overlooked or treated in a cursory fashion when developing SOM models. The purpose of this paper is to review current knowledge of SOM dynamics within the framework of a newly proposed soil C saturation concept.Initially,we distinguish SOM that is protected against decomposition by various mechanisms from that which is not protected from decomposition.Methods of quantification and characteristics of three SOM pools defined as protected are discussed.Soil organic matter can be:(1)physically stabilized,or protected from decomposition,through microaggregation,or(2)intimate association with silt and clay particles, and(3)can be biochemically stabilized through the formation of recalcitrant SOM compounds.In addition to behavior of each SOM pool,we discuss implications of changes in land management on processes by which SOM compounds undergo protection and release.The characteristics and responses to changes in land use or land management are described for the light fraction(LF)and particulate organic matter(POM).We defined the LF and POM not occluded within microaggregates(53–250µm sized aggregates as unprotected.Our conclusions are illustrated in a new conceptual SOM model that differs from most SOM models in that the model state variables are measurable SOM pools.We suggest that physicochemical characteristics inherent to soils define the maximum protective capacity of these pools,which limits increases in SOM(i.e.C sequestration)with increased organic residue inputs.IntroductionMost current models of SOM dynamics assumefirst-order kinetics for the decomposition of various con-ceptual pools of organic matter(McGill,1996;Paus-tian,1994),which means that equilibrium C stocks are linearly proportional to C inputs(Paustian et al., 1997).These models predict that soil C stocks can, in theory,be increased without limit,provided that C inputs increase without limit,i.e.there are no as-sumptions of soil C saturation.While these models have been largely successful in representing SOM dynamics under current conditions and management practices(e.g.Parton et al.,1987,1994;Paustian et ∗FAX No:+1-970-491-1965.E-mail:johan@ al.,1992;Powlson et al.,1996),usually for soils with low to moderate C levels(e.g.<5%),there is some question of their validity for projecting longer term SOM dynamics under scenarios of ever increasing C inputs(e.g.Donigian et al.,1997).Such scenarios are particularly relevant with the development of new technology designed to promote soil C sequestration through increasing plant C inputs.Native soil C levels reflect the balance of C inputs and C losses under native conditions(i.e.productivity, moisture and temperature regimes),but do not neces-sarily represent an upper limit in soil C stocks.Empir-ical evidence demonstrates that C levels in intensively managed agricultural and pastoral ecosystems can ex-ceed those under native conditions.Phosphorous fer-tilization of Australian pasture soils can increase soil C by150%or more relative to the native condition156(Barrow,1969;Ridley et al.,1990;Russell1960). Soil C levels under long-term grassland(‘near native’) vegetation have also been exceeded in high productiv-ity mid-western no-tillage(NT)systems(Ismail et al., 1994)as well as in sod plots with altered vegetation (Follett et al.,1997).Hence,native soil C levels may not be an appropriate measure of the ultimate C sink capacity of soils.There are several lines of evidence that suggest the existence of a C saturation level based on physiochem-ical processes that stabilize or protect organic com-pounds in soils.While many long-termfield experi-ments exhibit a proportional relationship between C inputs and soil C content across treatments(Larson et al.,1972;Paustian et al.,1997),some experiments in high C soils show little or no increase in soil C content with two to three fold increases in C inputs (Campbell et al.,1991;Paustian et al.,1997;Solberg et al.,1997).Various physical properties(e.g.silt plus clay content and microaggregation)of soil are thought to be involved in the protection of organic materials from decomposer organism.However,these proper-ties and their exerted protection seem to be limited by their characteristics(e.g.surface area),which is con-sistent with a saturation phenomenon(Hassink,1997; Kemper and Koch,1966).A number of soil organic matter models have been developed in the last30years.Most of these mod-els represent the heterogeneity of SOM by defining several pools,typically three tofive,which vary in their intrinsic decay rates and in the factors which control decomposition rates(see reviews by McGill, 1996;Parton,et al.,1994;Paustian,1994).Alternat-ive formulations,whereby specific decomposition rate varies as a function of a continuous SOM quality spec-trum(i.e.instead of discrete pools),have also been developed(e.g.Bosatta and Agren,1996).However, in either case,the representation of the model pools (or quality spectrum)is primarily conceptual in nature. While such models can be successfully validated us-ing measurements of total organic carbon and isotopic ratios of total C(e.g.Jenkinson and Rayner,1977), the individual pools are generally only loosely associ-ated with measurable quantities obtained with existing analytical methods.Consequently,it is not straight-forward to falsify or test the internal dynamics of C transfers between pools and changes in pool sizes of the current SOM models with conceptual pool defin-itions because a direct comparison to measured pool changes is not possible.A closer linkage between theoretical and measur-able pools of SOM can be made by explicitly defining model pools to coincide with measurable quantities or by devising more functional laboratory fractiona-tion procedures or both.The phrases‘modeling the measurable’and‘measuring the modelable’have been coined as representing the two approaches towards a closer reconciliation between theoretical and experi-mental work on SOM(Christensen,1996;Elliott et al.,1996).Various attempts have been made to correlate ana-lytical laboratory fractions with conceptual model pools,with limited success.Motavalli et al.(1994) compared laboratory measurements of C mineraliza-tion with simulations by the Century model(Parton et al.,1994)for several tropical soils.When the active and slow pools in the model were initialized using laboratory determinations of microbial+soluble C for the active pool and light fraction for the slow pool,C mineralization was consistently underestim-ated,although all fractions were highly significantly correlated to C mineralization in a regression ana-lysis.Magid et al.(1996)unsuccessfully attempted to trace14C labeled plant materials using three size-density fractionation methods to define an‘active’pool.Metherell(1992)found that the slow pool in Century was much larger than the particulate organic matter(POM)fraction isolated from a Haplustoll by Cambardella and Elliott(1992).However,Balesdent (1996)found that POM isolated after mild disrup-tion corresponds to the plant structural compartment (RPM)of the Rothamsted carbon model(Jenkinson and Rayner,1997).Acid hydrolysis has been used to estimate Century’s passive C pool(Paul et al.,1997a; Trumbore,1993),but it seems to slightly overestimate the size(Paul et al.,1997a;Trumbore,1993),though not the C turnover rate,of the passive pool(Trumbore, 1993).Nevertheless,Paul et al.(1999)used extended laboratory incubations in combination with acid hy-drolysis to define an active,slow and passive pool of C and were successful in modeling the evolution of CO2in thefield based on these pools.These studies suggest that attempting to measure the modelable has had minimal success to date.There have been a few recent attempts to more closely integrate models and measurements of physi-cochemically defined pools by‘modeling the measur-able’,although Elliott et al.(1996)and Christensen (1996)have presented conceptual models for this ap-proach.Arah(2000)proposed an approach based on analytically defined pools and measurements of13C157Figure1.The protective capacity of soil(which governs the silt-and clay protected C and microaggregate protected C pools),the biochemically stabilized C pool and the unprotected C pool define a maximum C content for soils.The pool size of each fraction is determined by their unique stabilizing mechanisms.and15N stable isotope tracers to derive parameters for a model with measurable pools.The approach con-siders all possible transformations between measured C and N pools and devises a system of equations using observed changes in total C and N and13C and15N for each fraction to solve all model unknowns.Necessary requirements of such an approach are that the analyt-ical fractions are distinct and together account for the total carbon inventory.The objective of this review paper is to summar-ize current knowledge on SOM dynamics and sta-bilization and to synthesize this information into a conceptual SOM model based on physicochemically defined SOM pools.This new model defines a soil C-saturation capacity,or a maximum soil C storage potential,determined by the physicochemical proper-ties of the soil.We propose that the conceptual model developed from this knowledge may form the basis for a simulation model with physicochemically measur-able SOM pools as state variables rather than with the biologically defined pools by Paul et al.(1999).Protected SOM:Stabilization mechanisms, characteristics,and dynamicsThree main mechanisms of SOM stabilization have been proposed:(1)chemical stabilization,(2)phys-ical protection and(3)biochemical stabilization (Christensen,1996;Stevenson,1994).Chemical sta-bilization of SOM is understood to be the result of the chemical or physicochemical binding between SOM and soil minerals(i.e.clay and silt particles).Indeed, many studies have reported a relationship between stabilization of organic C and N in soils and clay or silt plus clay content(Feller and Beare,1997; Hassink,1997;Ladd et al.,1985;Merckx et al., 1985;Sorensen,1972).In addition to the clay con-tent,clay type(i.e.2:1versus1:1versus allophanic clay minerals)influences the stabilization of organic C and N(Feller and Beare,1997;Ladd et al.1992; Sorensen,1972;Torn et al.,1997).Physical protection by aggregates is indicated by the positive influence of aggregation on the accumulation of SOM(e.g.Ed-wards and Bremner,1967;Elliott,1986;Jastrow, 1996;Tisdall and Oades,1982;Six et al.,2000a). Aggregates physically protect SOM by forming phys-ical barriers between microbes and enzymes and their substrates and controlling food web interactions and consequently microbial turnover(Elliott and Coleman, 1988).Biochemical stabilization is understood as the stabilization of SOM due to its own chemical com-position(e.g.recalcitrant compounds such as lignin and polyphenols)and through chemical complexing processes(e.g.condensation reactions)in soil.For our analyses,we divide the protected SOM pool into three pools according to the three stabilization mechanisms described(Figure1).The three SOM pools are the silt-and clay-protected SOM(silt and clay defined as <53µm organomineral complexes),microaggregate-protected SOM(microaggregates defined as53–250µm aggregates),and biochemically protected SOM. Chemical stabilization:Silt-and clay-protected SOM The protection of SOM by silt and clay particles is well established(Feller and Beare,1997;Hassink, 1997;Ladd et al.,1985;Sorensen,1972).Hassink (1997)examined the relationship between SOM frac-tions and soil texture and found a relationship between the silt-and clay-associated C and soil texture,though he did notfind any correlation between texture and amount of C in the sand-sized fraction(i.e.POM C).Based on thesefindings,he defined the capacity158Table1.Regression equations relating silt plus clay proportion to silt and clay associated CSize class a Ecosystem Intercept Slope r20–20µm Cultivated 4.38±0.68b0.26±0.010.41Grassland 2.21±1.940.42±0.080.44Forest−2.51±0.550.63±0.010.550–50µm Cultivated7.18±3.040.2±0.040.54Grassland16.33±4.690.32±0.070.35Forest16.24±6.010.24±0.080.35Size class Clay type Intercept Slope r20–20µm1:1 1.22±0.370.30±0.010.742:1 3.86±0.490.41±0.010.390–50µm1:1 5.5±5.930.26±0.130.382:114.76±2.370.21±0.030.07a Two size classes for silt and clay were reported in the literature.b Value±95%confidence interval.of soil to preserve C by its association with silt and clay particles.Studies investigating the retention of specific microbial products(i.e.amino sugars)cor-roborate the proposition of Hassink(1997)that C associated with primary organomineral complexes arechemically protected and the amount of protection in-creased with an increased silt plus clay proportion of the soil(Chantigny et al.,1997;Guggenberger et al., 1999;Puget et al.,1999;Sorensen,1972).Puget et al.(1999)reported an enrichment of microbial derived carbohydrates in the silt plus clay fraction compared to the sand fraction of no-tilled and conventional tilled soils.However,the amount stabilized by silt and clay differs among microbial products.For example,Gug-genberger et al.(1999)reported a higher increase of glucosamine than muramic acid under no-tillage at sites with a high silt plus clay content.A reexamin-ation of the data presented by Chantigny et al.(1997) leads to the observation that the glucosamine/muramic acid ratio was only higher in perennial systems com-pared to annual systems in a silty clay loam soil and not in a clay loam soil.The silty clay loam soil had a higher silt plus clay content.We expanded the analysis of Hassink(1997)of the physical protection capacity for C associated with primary organomineral complexes(Figure2)across ecosystems(i.e.forest,grassland,and cultivated sys-tems),clay types(i.e.1:1versus2:1),and size ranges for clay and silt(0–20µm and0–50µm;see Ap-Figure 2.The relationship between silt+clay content(%)and silt+clay associated C(g silt+clay C kg−1soil)for grassland,forest and cultivated ecosystems.A differentiation between1:1clay and 2:1clay dominated soils is also made.The relationships indicate a maximum of C associated with silt and clay(i.e.C saturation level for the clay and silt particles),which differs between forest and grassland ecosystems and between clay types.Two size boundaries for silt+clay were used(A)0–20µm and(B)0–50µm. pendices for details).Following the methodology of Hassink(1997)we performed regressions(Figure2 and Table1)between the C content associated with silt and clay particles(g C associated with silt and clay particles kg−1soil;Y axis)and the proportion of silt and clay particles(g silt plus clay g−1soil;X axis).All regressions were significant(P<0.05)and comparison of regression lines revealed that the influ-ence of soil texture on mineral-associated C content differed depending on the size range used for clay and silt particles.Consequently,we did regressions for two different size classes of silt and clay particles(i.e.0–20µm and0–50µm;Figure2and Table1).The intercept for the0–50µm silt and clay particles was significantly higher than for the0–20µm silt and clay particles(Table1).This difference in intercept was159probably a result of the presence of larger sized(20–50µm)silt-sized aggregates in the0–50µm than in the 0–20µm silt and clay particles.These larger silt-sized aggregates have more C per unit material because ad-ditional C binds the primary organomineral complexes into silt-sized aggregates(Tisdall and Oades,1982). However the difference in intercept might also be the result of POM particles of the size20–50µm as-sociated with the0–50µm fraction(Turchenek and Oades,1979).Intercepts for cultivated and forest eco-systems were significantly different for the0–50µm particles,but were only marginally significantly dif-ferent(P<0.06)for the0–20µm particles.Slopes for grassland soils(0–20µm particles)were signi-ficantly different than those for forest and cultivated soils.The differences between grasslands and cultiv-ated lands are likely due to differences in input and disturbance,which causes a release of SOM and con-sequently increased C availability for decomposition. An explanation for the significantly different slopes for grassland and forest soils(Table1)is not imme-diately apparent.Especially that the slope is higher for forest than grassland slopes.This is in contrast to the suggestion that grassland-derived soils have a higher potential of C stabilization than forest-derived because of their higher base saturation(Collins et al.,2000; Kononova,1966).Consequently,this difference in C stabilization by silt and clay particles between forest and grassland systems should be investigated further.In contrast to Hassink(1997),we found signific-antly different relationships for1:1clays versus2:1 clays regressions and for the cultivated versus grass-land regressions(Figure2and Table1)for the0–20µm particles.The effect of clay type was also signific-ant for the0–50µm particles.This lower stabilization of C in1:1clay dominated soils is probably mostly re-lated to the differences between the clay types(see be-low).However,the effect of climate can not be ignored in this comparison because most1:1clay dominated soils were located in(sub)tropical regions.The higher temperature and moisture regimes in(sub)tropical re-gions probably also induce a faster decomposition rate and therefore contributes to the lower stabilization of C by the1:1clays.Nevertheless we believe that the type of clay plays an important role because different types of clay(i.e.1:1and2:1clays)have substantial differences in CEC and specific surface(Greenland, 1965)and should,consequently,have different ca-pacities to adsorb organic materials.In addition,Fe-and Al-oxides are most often found in soils domin-ated by1:1minerals and are strongflocculants.By being strongflocculants,Fe-and Al-oxides can re-duce even further the available surface for adsorption of SOM.We are not certain why soils examined by Hassink(1997)did not follow this reduced capacity to adsorb organic materials;few soils dominated by 1:1clays,however,were included in the data set used by Hassink(1997)and most of them had a low car-bon content.Nevertheless,the difference between the two studies might also be a result of the contrast-ing effect the associated Fe-and Al-oxides can have. The strongflocculating oxides can reduce available surface(see above)but they might also co-flocculate SOM and consequently stabilize it.Therefore,it ap-pears that mechanisms with contrasting effects on SOM stabilization exist and the net effect still needs to be investigated.The different regression lines for grassland and cultivated systems are in accordance with Feller et al.(1997).They also found a signific-ant lower slope for the regression line between the amount of0–2µm particles and the C contained in the0–2µm fraction of cultivated soils compared to non-cultivated soils.The lack of influence of cultiv-ation on the silt and clay associated C observed by Hassink(1997)was probably a result of the low pro-portion of silt and clay and high SOM contents of the soils used.The silt-and clay-associated C formed a small fraction of the total C in his soils.Consequently, sand-associated C accounted for the majority of total soil C.Given this dominance of sand-associated C and its greater sensitivity to cultivation than silt-and clay-associated C(Cambardella and Elliott,1992),in which C is transferred from the sand associated fraction to the silt-and clay-associated fractions during decom-position(Guggenberger et al.,1994),a loss of silt-and clay-associated C upon cultivation is likely to be minimal.In summary,we found,as Hassink(1997)did,a direct relationship between silt plus clay content of soil and the amount of silt-and clay-protected soil C, indicating a saturation level for silt and clay associated C.This relationship was different between different types of land use,different clay types,and for differ-ent determinations of silt plus clay size class.Also, the silt-and clay-associated soil organic matter was reduced by cultivation.Physical protection:Microaggregate-protected SOM The physical protection exerted by macro-and/or mi-croaggregates on POM C is attributed to:(1)the compartmentalization of substrate and microbial bio-160mass(Killham et al.,1993;van Veen and Kuikman, 1990),(2)the reduced diffusion of oxygen into macro-and especially microaggregates(Sexstone et al.,1985) which leads to a reduced activity within the aggregates (Sollins et al.,1996),and(3)the compartmentalization of microbial biomass and microbial grazers(Elliott et al.,1980).The compartmentalization between sub-strate and microbes by macro-and microaggregates is indicated by the highest abundance of microbes on the outer part of the aggregates(Hattori,1988)and a substantial part of SOM being at the center of the aggregates(Elliott and Coleman,1988;Golchin et al., 1994).In addition,Bartlett and Doner(1988)repor-ted a higher loss of amino acids by respiration from the aggregate surfaces than from within aggregates. Priesack and Kisser-Priesack(1993)showed that the rate of glucose utilization decreased with distance into the aggregate.The inaccessibility of substrate for mi-crobes within aggregates is due to pore size exclusion and related to the water-filled porosity(Killham et al., 1993).Many studies have documented a positive influ-ence of aggregation on the accumulation of SOM(An-gers et al.,1997;Besnard et al.,1996;Cambardella and Elliott,1993;Franzluebbers and Arshad,1997; Gale et al.,2000;Golchin et al.,1994,1995;Jastrow, 1996;Monreal and Kodama,1997;Paustian et al., 2000;Puget et al.,1995,1996;Six et al.,1998,1999, 2000a).Cultivation causes a release of C by break-ing up the aggregate structures,thereby increasing availability of C.More specifically,cultivation leads to a loss of C-rich macroaggregates and an increase of C-depleted microaggregates(Elliott,1986;Six et al.,2000a).The inclusion of SOM in aggregates also leads to a qualitative change of SOM.For example, Golchin et al.(1994)reported significant differences in chemical structure between the free and occluded (i.e.within aggregates)light fraction.The occluded light fraction had higher C and N concentrations than the free light fraction and contained more alkyl C(i.e. long chains of C compounds such as fatty acids,lipids, cutin acids,proteins and peptides)and less O-alkyl C(e.g.carbohydrates and polysaccharides).These data suggest that during the transformation of free into intraaggregate light fraction there is a selective decomposition of easily decomposable carbohydrates (i.e.O-alkyl C)and preservation of recalcitrant long-chained C(i.e.alkyl C)(Golchin et al.,1994).Golchin et al.(1995)also found that cultivation decreased the O-alkyl content of the occluded SOM.They sugges-ted that this difference is a result of the continuous disruption of aggregates,which leads to a faster min-eralization of SOM and a preferential loss of readily available O-alkyl C.Hence,the enhanced protection of SOM by aggregates in less disturbed soil results in an accumulation of more labile C than would be maintained in a disturbed soil.Recent studies indicate that the macroaggreg-ate(>250µm)structure exerts a minimal amount of physical protection(Beare et al.,1994;Elliott, 1986;Pulleman and Marinissen,2001),whereas SOM is protected from decomposition in free(i.e.not within macroaggregates)microaggregates(<250µm) (Balesdent et al.,2000;Besnard et al.,1996;Skjem-stad et al.,1996)and in microaggregates within mac-roaggregates(Denef et al.,2001;Six et al.,2000b). Beare et al.(1994)and Elliott(1986)found an increase in C mineralization when they crushed macroaggreg-ates,but the increase in mineralization only accounted for1–2%of the C content of the macroaggregates.In addition,no difference in C mineralization between crushed and uncrushed macroaggregates has been ob-served(Pulleman and Marinissen,2001).In contrast, C mineralization of crushed free microaggregates was three to four times higher than crushed macroaggreg-ates(Bossuyt et al.,2002).Gregorich et al.(1989) observed a substantial higher C mineralization when microaggregates within the soil were disrupted than when lower disruptive energies were used that did not break up microaggregates.Jastrow et al.(1996),us-ing13C natural abundance technique,calculated that the average turnover time of C in free microaggreg-ates was412yr,whereas the average turnover time for macroaggregate associated C was only140yr in the surface10cm.These studies clearly indicate that C stabilization is greater within free microaggregates than within macroaggregates.Further corroborating evidence for the crucial role microaggregates play in C sequestration were reported by Angers et al.(1997), Besnard et al.(1996),Gale et al.(2000)and Six et al.(2000b).Angers et al.(1997)found in afield in-cubation experiment with13C and15N labeled wheat straw that wheat-derived C was predominantly stored and stabilized in free microaggregates.Gale et al. (2000)reported similar C stabilization within free mi-croaggregates in an incubation study with14C-labeled root material.Upon conversion of forest to maize cul-tivation,Besnard et al.(1996)found a preferential accumulation of maize-and forest-derived POM-C in microaggregates compared to other soil fractions. Six et al.(1999)observed a decrease infine intra-macroaggregate-POM(i.e.53–250µm sized POM161(fine iPOM)predominantly stabilized in microaggreg-ates within macroaggregates(Six et al.,2000b))under plough tillage compared to no-till.However,there was no difference in coarse intra-macroaggregate POM (i.e.250–2000µm POM not stabilized by the micro-aggregates within macroaggregates)between tillage systems at three of the four sites studied.They con-cluded that the incorporation and stabilization offine POM-C into microaggregates within macroaggregates and free microaggregates under no-tillage is a dom-inant factor for protection of thefine-sized fraction of POM.Nevertheless,the dynamics of macroaggreg-ates are crucial for the sequestration of C because it influences the formation of microaggregates and the sequestration of C within these microaggregates(Six et al.,2000b).That is,rapid turnover of macroaggreg-ates reduces the formation of microaggregates within macroaggregates and the resulting stabilization of C within these microaggregates(Six et al.,1998,1999, 2000b).Though the incorporation of POM into microag-gregates(versus bonding to clay surfaces;i.e.chem-ical mechanism)seems to be the main process for protection of POM,the clay content and type of soil exert an indirect influence on the protection of POM-C by affecting aggregate dynamics.Franzluebbers and Arshad(1997)suggested that physical protec-tion of POM within aggregates increases with clay content since mineralization of POM-C relative to whole-SOM-C after dispersion and aggregation both increased with increasing clay content(Franzluebbers and Arshad,1996).Different clay types lead to differ-ent mechanisms involved in aggregation(Oades and Waters,1991)and will therefore influence differently the protection of POM through microaggregation. Within the2:1clay minerals,clay minerals with a high CEC and larger specific surface,such as montmoril-lonite and vermiculite,have a higher binding potential than clay minerals with a lower CEC and smaller spe-cific surface,such as illite(Greenland,1965).In con-trast to the2:1minerals,kaolinite and especially Fe-and Al-oxides have a highflocculation capacity due to electrostatic interactions through their positive charges (Dixon,1989;Schofield and Samson,1954).Even though,different mechanisms prevail in soils with dif-ferent clay types,soils seem to have a maximum level of aggregate stability.Kemper and Koch(1966)ob-served that aggregate stability increased to a maximum level with clay content and free Fe-oxides content. Since the physical protection of POM seems to be mostly determined by microaggregation,we hypothes-ize that the maximum physical protection capacity for SOM is determined by the maximum microaggrega-tion,which is in turn determined by clay content,clay type.Biochemical stabilization:Biochemically-protected SOMIn this review,a detailed description of the influ-ence of biochemical stabilization on SOM dynamics will not be given,we refer to an excellent review on this subject by Cadisch and Giller(1997).Nev-ertheless,biochemical stabilization of SOM needs to be considered to define the soil C-saturation level within a certain ecosystem(Figure1).Biochemical stabilization or protection of SOM occurs due to the complex chemical composition of the organic mater-ials.This complex chemical composition can be an inherent property of the plant material(referred to as residue quality)or be attained during decomposition through the condensation and complexation of decom-position residues,rendering them more resistant to subsequent decomposition.Therefore the third pool in our model(Figure1)is a SOM pool that is stabilized by its inherent or acquired biochemical resistance to decomposition.This pool is akin to that referred to as the‘passive’SOM pool(Parton et al.,1987)and its size has been equated to the non-hydrolyzable frac-tion(Leavitt et al.,1996;Paul et al.,1995;Trumbore 1993).Using14C dating,it has been found that,in the surface soil layer,the non-hydrolyzable C is ap-proximately1300years older than total soil C(Paul et al.,1997a,2001).Several studies have found that the non-hydrolyzable fraction in temperate soils includes very old C(Anderson and Paul,1984;Paul et al., 1999;Trumbore,1993;Trumbore et al.,1996)and acid hydrolysis removes proteins,nucleic acids,and polysaccharides(Schnitzer and Khan,1972)which are believed to be more chemically labile than other C compounds,such as aromatic humified compon-ents and wax-derived long chain aliphatics(Paul et al.,1997a).The stabilization of this pool and con-sequent old age is probably predominantly the result of its biochemical composition.However,Balesdent (1996)did notfind any great differences in dynam-ics between the non-hydrolyzable and hydrolyzable C fraction and therefore questioned the relationship between biodegradability and hydrolyzability.Never-theless,we chose the hydrolysis technique to differen-tiate an older and passive C pool,because we think it is the simplest and best available technique to define。
Soilorganicmatteranddegradation:土壤有机质降解
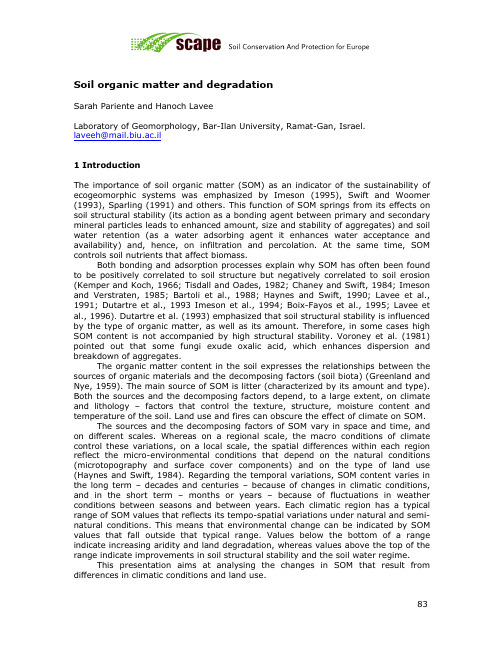
Soil organic matter and degradationSarah Pariente and Hanoch LaveeLaboratory of Geomorphology, Bar-Ilan University, Ramat-Gan, Israel.***************.ac.il1 IntroductionThe importance of soil organic matter (SOM) as an indicator of the sustainability of ecogeomorphic systems was emphasized by Imeson (1995), Swift and Woomer (1993), Sparling (1991) and others. This function of SOM springs from its effects on soil structural stability (its action as a bonding agent between primary and secondary mineral particles leads to enhanced amount, size and stability of aggregates) and soil water retention (as a water adsorbing agent it enhances water acceptance and availability) and, hence, on infiltration and percolation. At the same time, SOM controls soil nutrients that affect biomass.Both bonding and adsorption processes explain why SOM has often been found to be positively correlated to soil structure but negatively correlated to soil erosion (Kemper and Koch, 1966; Tisdall and Oades, 1982; Chaney and Swift, 1984; Imeson and Verstraten, 1985; Bartoli et al., 1988; Haynes and Swift, 1990; Lavee et al., 1991; Dutartre et al., 1993 Imeson et al., 1994; Boix-Fayos et al., 1995; Lavee et al., 1996). Dutartre et al. (1993) emphasized that soil structural stability is influenced by the type of organic matter, as well as its amount. Therefore, in some cases high SOM content is not accompanied by high structural stability. Voroney et al. (1981) pointed out that some fungi exude oxalic acid, which enhances dispersion and breakdown of aggregates.The organic matter content in the soil expresses the relationships between the sources of organic materials and the decomposing factors (soil biota) (Greenland and Nye, 1959). The main source of SOM is litter (characterized by its amount and type). Both the sources and the decomposing factors depend, to a large extent, on climate and lithology – factors that control the texture, structure, moisture content and temperature of the soil. Land use and fires can obscure the effect of climate on SOM.The sources and the decomposing factors of SOM vary in space and time, and on different scales. Whereas on a regional scale, the macro conditions of climate control these variations, on a local scale, the spatial differences within each region reflect the micro-environmental conditions that depend on the natural conditions (microtopography and surface cover components) and on the type of land use (Haynes and Swift, 1984). Regarding the temporal variations, SOM content varies in the long term – decades and centuries – because of changes in climatic conditions, and in the short term – months or years – because of fluctuations in weather conditions between seasons and between years. Each climatic region has a typical range of SOM values that reflects its tempo-spatial variations under natural and semi-natural conditions. This means that environmental change can be indicated by SOM values that fall outside that typical range. Values below the bottom of a range indicate increasing aridity and land degradation, whereas values above the top of the range indicate improvements in soil structural stability and the soil water regime.This presentation aims at analysing the changes in SOM that result from differences in climatic conditions and land use.2 Research sitesThe research was carried out in several research sites, representing Mediterranean (sub-humid), semi-arid, mildly arid and arid climates along a climatic transect, running from the Judean mountains (mean annual rainfall 700 mm, and annual meantemperature 17°C) to the Dead Sea (mean annual rainfall under 100 mm, and annualmean temperature 23°C) (Figure 1). Five research stations were established onhillslopes having similar topographic (azimuth 135-150° and gradients of 11-14°) and lithological (hard calcareous bedrock) conditions. The climatic characteristics (Table 1) vary widely among the sites except for sites MAB and MAL, in which they are the same. However, these two sites differ from each other in their surface cover characteristics: MAB has fewer shrubs and annuals but more rock fragments than MAL.Figure 1. Locations of the study sites (mean annual rainfall in mm, is indicated by isohyets).Table 1. The main ecogeomorphological characteristics of the research sites.Research site Meanannualtemperature(°C )Meanannual rainfall(mm)Soil type Vegetation coverin March 2000(%)Giv’at Ye’arim (GIV) 17 620 BrownTerraRossa85Ma’ale Adumim (MAL) 19 330 BrownRendzina40Ma’ale Adumim (MAB) 19 330 BrownRendzina30Mishor Adumin(MIS)20 260 Pale brown lithosol 30KALIA (KAL) 23 120 Very pale gypsicdesert lithosol103. MethodAt four sites, GIV, MAL, MIS and KAL, soil samples were taken four times a year, inJanuary, March, May and September, from 1992 through 1993 and 1994 and in April and August 2000. In the last two months soil samples were taken in site MAB too. Ateach of the sites, in each season, four to eight points were sampled in open areas between shrubs. At each point soil samples were taken from two soil depths: 0-2 cm, and 2-10 cm. The organic matter content was determined by the wet combustion method (Head, 1984).Data were statistically evaluated by analysis of variance with SPSS 10 for Windows (SPSS Inc. Chicago, USA). Tukeys test, at α=0.05 level of significance, was used.4 Results and discussion4.1 Effect of climate conditionsComparison between the sites along the climatic transect shows that, except for siteMAB, SOM increased significantly in both 0-2 cm and 2-10 cm, from the arid site, KAL, through the mildly arid site, MIS, and the semi-arid site, MAL, to the Mediterranean site, GIV. This increase is accounted for by differences between the climatic zones, in the relationships between the sources of the soil organic matter – mainly vegetation – and the decomposing factors, i.e., micro organisms. The arid zone is characterized by a low vegetation cover (Table 1), which exists for a short time, so that the sources of organic matter are limited in both quantity and availability. In this zone the conditions that favour micro organism activity in the soil also prevail for a short time, and are limited to the winter and spring, when the soil moisture and temperature do not restrict such activity (Li and Sarah, 2003a,b). Also, the high salinity that characterizes this zone inhibits microbial biomass (Sarah, 2001). The Mediterranean zone is characterized by a high vegetation cover which exists both in the winter and in the summer, and by soil moisture content and temperatures suitable for biotic activities during a large proportion of the year. These characteristics result in a high organic matter content in the soil. Most of this SOM is in the form of polymers that facilitate the establishment of strong and flexible connections between the inorganic soil particles that form the aggregates, as a result of which the disintegration and dispersion of the aggregates during wetting and drying are relatively low (Emerson et al., 1986).Table 2. Soil organic matter at different depths at the study sites. Means in the row within one depth followed by different capital letters varied significantly at the 0.05 probability level. Means in the column within one site followed by different letters varied significantly at the 0.05 probability level.Depth/Siten KAL76MIS76MAB16MAL76GIV760-2 cm a 1.46 E a 2.56 C a 2.12 D a 3.77 B a 6.15 A 2-10 cm b 1.07 D b 2.05 C a 1.89 C b 2.96 B b 4.39 A4.2 Effect of land useFigure 2 and Table 2 show significant differences, in each of the soil depths, betweenthe two semi-arid sites, MAL and MAB, in spite of the fact that they are under the same climatic conditions. The SOM content in site MAB was significantly lower thanthat in site MAL in the two depths. The SOM content in the 0-2 cm depth in site MAB was significantly lower even than that in site MIS, which is more arid.Figure 2. Soil organic matter content variations along the climatic gradient.Measurements of SOM in other natural semi-arid sites with similar topographic andlithological conditions show similar values to those of site MAL. The conclusion is that while SOM values in MAL represent the semi-arid zone under natural/semi-naturalconditions, the values in site MAB express a deviation that indicates land degradation, probably because of intensive human interference, such as overgrazing and/or the establishment of nomad settlement (tents). This conclusion is strengthened by the surface cover characteristics at this site, i.e., relatively low density of shrubs and high density of rock fragments. Furthermore, Bedouin encampments are still seen in the neighborhood.Comparison between the two soil depths shows that whereas in all sites SOM inthe 0-2 cm was significantly higher than that in 2-10 cm, the difference in site MAB was small and not significant (Table 2). No intensive human interference was observed in site MAB in the last 12 years. This means that site MAB went through a severe degradation and did not recover yet.To sum up, significant deviations from the typical expected SOM values in both the regional scale and the soil profile scale can be used as indices of land degradation. This emphasizes the importance of field long term monitoring. ReferencesBartoli, F., Philippy, R. and Burtin, G., 1988. Aggregation in soils with small amounts of swelling clays.Aggregate stability. J. Soil Science 39, 593-616.Boix-Fayos, C., Soriano, M.D., Tiemessen, I.R., Calvo-Cases, A. and Imeson, A.C., 1995. Properties and erosional response of soils in a degraded ecosystem in Crete (Greece). Environmental Monitoring Assessment 37, 79-92.Chaney, K. and Swift, R.S., 1984. The influence of organic matter on aggregate stability in some British soils. J. Soil Science 35, 223-230.Dutartre, Ph., Bartoli, F., Andreux, F., Portal, J.M. and Ange, A., 1993. Influence of content and nature of organic matter on the structure of some sandy soils from West Africa. Geoderma 56, 459-478. Emerson, W.W., Foster, R.C. and Oades, J.M., 1986. Organo-Mineral Complexes in Relation to Soil Aggregation and Structure. In: Huang, P. M. and Schnitzer, M. (Eds.), Interactions of Soil Minerals with Natural Organics and Microbes, Soil Science Society of America Spec. Pub. no. 17, 521-548. Greenland, D.J. and Nye, P.H., 1959. Increase in the carbon and Nitrogen contents of tropical soils under natural fallows. J. Soil Science 10, 284-299.Haynes, R.J. and Swift, R.S., 1990. Stability of aggregates in relation to organic constituents and soil water content. J. Soil Science 41, 73-83.Head, K.H., 1984. Manual of Soil Laboratory Testing, 1, Soil Classification and Compaction Tests. ELE International Ltd. Fentech Press, London.Imeson, A.C., 1995. The physical, chemical and biological degradation of the soil. In Fantechi, R., Peter,D., Balabanis, P. and Rubio, J.L. (Eds.): Desertification in a European Context: Physical and socio-economic aspects. Proceedings of the European School 0f Climatology and Natural Hazards course, Alicante, 1993, 399-409.Imeson, A.C. and Verstraten J.M., 1985. The erodibility of highly calcareous soil material from southern Spain. Catena 12, 291-306.Imeson, A.C., Perez-Trejo, F., Lavee, H. and Calvo-Cases, A., 1994. Modelling and exploring the impact of climate change on ecosystem degradation, hydrology and land use along a transect across the Mediterranean. In Troen, I. (Ed.), Global Change: Climatic Change and Climatic Change Impacts.Proceedings Copenhagen Symposium, September 1993, European Commission, EUR 15921 EN, 173-185.Kemper, W.D. and Koch, E.J., 1966. Aggregate stability of soils from Western United States and Canada.USDA Tech. Bull. 1355, 52 p.Lavee, H, Imeson, A.C., Sarah, P. and Benyamini, Y., 1991. The response of soils to simulated rainfall along a climatological gradient in an arid and semi-arid region. Catena 19, 19-37.Lavee, H., Sarah, P. and Imeson, A.C., 1996. Aggregate stability dynamics as affected by soil temperature and moisture regimes. Geografiska Annaler 78A, 73-82.Li, X. and Sarah, H. 2003. Enzyme activities along a climatic transect in the Judean Desert. Catena (in press).Li, X. and Sarah, H. 2003. Arylsulfatase activity of soil microbial biomass along a Mediterranean-arid transect. Soil Biology and Biochemistry (in press).Sarah, P., 2001. Soluble salts dynamics in the soil under different climatic regions. Catena, 43: 307-321. Sparling, G.D., 1991. Organic matter carbon and microbial biomass carbon as indicators of sustainable land use. In Elliot, C. R., Latham, M. and Dumanski, J. (Eds.), Evaluation for Sustainable and Management in the Developing World. Vol. 2: Technical Papers. IBSRAM Proceedings No. 12. Bangkok, Thailand: IBSRAM.Swift, M.J. and Woomer, P., 1993. Organic matter and sustainability of agricultural systems: Definition and measurement. In Mulongoy, K. and Merckx, R. (Eds.), Soil Organic Matter Dynamics and Sustainability of Tropical Agriculture. John Wiley and Sons, 3-18.Tisdall, J.M. and Oades, J.M., 1982. Organic matter and water sTable aggregates in soil. J. Soil Science 33, 141-163.Voroney, R.P., van Veen, J.A. and Paul, E.A., 1981. Organic carbon dynamics in grassland soils. II. Model validation and simulation of the long-term effects of cultivation and rainfall erosion. Can. J. Soil Science 61, 211-224.。
污泥低温干化处理工艺流程
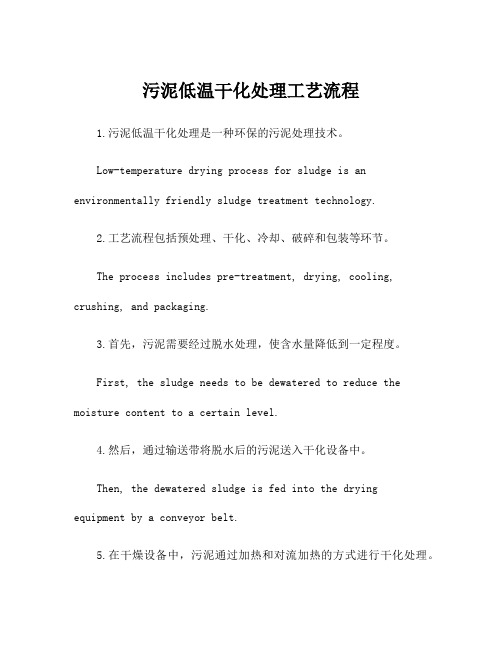
污泥低温干化处理工艺流程1.污泥低温干化处理是一种环保的污泥处理技术。
Low-temperature drying process for sludge is an environmentally friendly sludge treatment technology.2.工艺流程包括预处理、干化、冷却、破碎和包装等环节。
The process includes pre-treatment, drying, cooling, crushing, and packaging.3.首先,污泥需要经过脱水处理,使含水量降低到一定程度。
First, the sludge needs to be dewatered to reduce the moisture content to a certain level.4.然后,通过输送带将脱水后的污泥送入干化设备中。
Then, the dewatered sludge is fed into the drying equipment by a conveyor belt.5.在干燥设备中,污泥通过加热和对流加热的方式进行干化处理。
In the drying equipment, the sludge is dried by heating and convection heating.6.干化过程中,要保持适当的温度和湿度,以确保干燥效果。
During the drying process, the proper temperature and humidity should be maintained to ensure the drying effect.7.干化后的污泥经过冷却处理,使其温度适宜后进行下一步处理。
The dried sludge is cooled to an appropriate temperature for the next step of treatment.8.冷却后的污泥需要经过破碎处理,使其颗粒大小适合包装和运输。
土豆筷子实验 英文作文
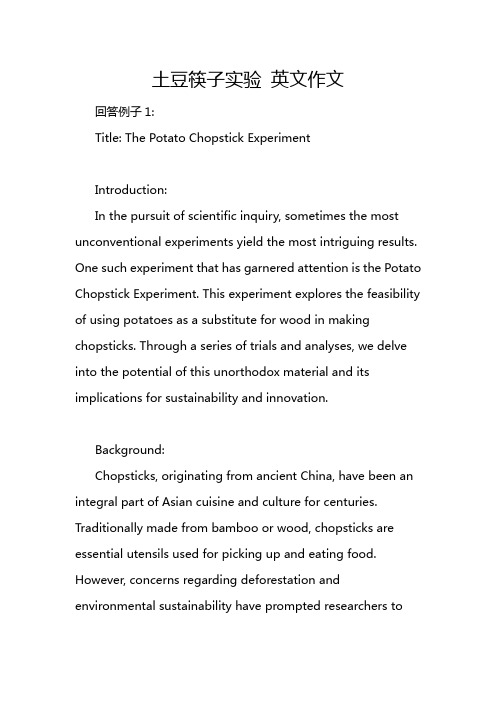
土豆筷子实验英文作文回答例子1:Title: The Potato Chopstick ExperimentIntroduction:In the pursuit of scientific inquiry, sometimes the most unconventional experiments yield the most intriguing results. One such experiment that has garnered attention is the Potato Chopstick Experiment. This experiment explores the feasibility of using potatoes as a substitute for wood in making chopsticks. Through a series of trials and analyses, we delve into the potential of this unorthodox material and its implications for sustainability and innovation.Background:Chopsticks, originating from ancient China, have been an integral part of Asian cuisine and culture for centuries. Traditionally made from bamboo or wood, chopsticks are essential utensils used for picking up and eating food. However, concerns regarding deforestation and environmental sustainability have prompted researchers toexplore alternative materials for chopstick production.Potatoes, a staple food in many cultures, offer a surprising potential as a substitute material. With their abundance, biodegradability, and renewability, potatoes present an intriguing possibility for sustainable chopstick production. The Potato Chopstick Experiment seeks to investigate whether potatoes possess the necessary properties to serve as a viable alternative to traditional wood or bamboo chopsticks.Experiment Design:The experiment begins by selecting high-quality potatoes and processing them into chopstick-sized rods. These potato rods are then subjected to various tests to evaluate their durability, flexibility, and usability compared to conventional chopsticks. Additionally, the experiment assesses the environmental impact of potato chopsticks by examining factors such as resource consumption and biodegradability.Results and Analysis:Initial trials reveal promising results, with potato chopsticks demonstrating adequate strength and flexibility forhandling food. However, challenges arise in achieving uniformity and consistency in the manufacturing process. Issues such as moisture content, density variations, and structural integrity pose obstacles to mass production.Further analysis indicates that while potato chopsticks may not be suitable for commercial use in their current form, they hold potential for innovation and refinement. By exploring different processing techniques and material enhancements, researchers can overcome existing limitations and optimize the performance of potato-based chopsticks.Implications:The Potato Chopstick Experiment underscores the importance of creativity and ingenuity in addressing environmental challenges. While the concept of potato chopsticks may seem unconventional, it embodies a spirit of exploration and resourcefulness in the quest for sustainable solutions.Beyond chopsticks, the findings of this experiment have broader implications for sustainable materials research. Byharnessing readily available resources like potatoes, we can reduce reliance on finite materials and minimize environmental impact. Moreover, the versatility of potatoes opens doors to diverse applications in various industries, from packaging to construction.Conclusion:In conclusion, the Potato Chopstick Experiment offers a fascinating glimpse into the intersection of tradition, innovation, and sustainability. While still in its experimental stages, the concept of potato chopsticks challenges conventional norms and inspires new possibilities for a greener future. Through continued research and collaboration, we can unlock the full potential of this humble tuber and pave the way for a more sustainable world.回答例子2:Title: The Potato Chopstick Experiment: A Journey of DiscoveryIntroduction:In the realm of scientific exploration, simplicity often unveils profound insights. The potato chopstick experimentexemplifies this principle, offering a fascinating avenue to delve into the realms of conductivity, circuitry, and the marvels of everyday materials. Through this experiment, we embark on a journey of discovery, unraveling the mysteries concealed within a humble potato and a pair of chopsticks.The Setup:To commence our expedition, assemble the following materials:- Two fresh potatoes- Two pairs of chopsticks (wooden or bamboo)- Copper and zinc electrodes (such as nails or coins)- Connecting wires with alligator clips- LED light bulbs (optional)- Multimeter (optional)Procedure:1. Select two potatoes of similar size and shape.2. Insert a copper electrode into one potato and a zinc electrode into the other, ensuring they don't touch.3. Position a chopstick in each potato, ensuring they touch the respective electrodes.4. Connect the chopsticks with wires and alligator clips to form a circuit.5. Observe any changes in the circuit, such as the illumination of LED bulbs or readings on a multimeter.Observations and Analysis:Upon completing the setup, the potato chopstick experiment unfolds its marvels. Despite its unassuming appearance, the potato serves as an electrolyte, facilitating the flow of electrons between the electrodes. The chopsticks, acting as conductors, allow this flow to occur, completing the circuit.The potato's role in this experiment is critical. As a starchy vegetable with high moisture content, it conducts electricity due to the ions present in its juice. When the chopsticks penetrate the potato, they absorb this moisture, enabling the flow of electrons along their length. The electrodes, made of different metals, create a potential difference, driving the electron flow through the circuit.Moreover, the LED bulbs serve as visual indicators of thecircuit's functionality. When the circuit is complete and current flows unhindered, the bulbs illuminate, demonstrating the conversion of chemical energy within the potato into electrical energy powering the bulbs. Alternatively, a multimeter provides quantitative measurements of voltage, current, and resistance, further enriching our understanding of the system's behavior.Implications and Further Exploration:Beyond its educational value, the potato chopstick experiment harbors significant implications in various domains. In the realm of sustainable energy, it underscores the potential of bio-electrochemical systems, where organic materials like potatoes could serve as sources of renewable energy. By harnessing the natural conductivity of organic matter, researchers envision novel approaches to powering low-energy devices and sensors in remote orresource-constrained environments.Furthermore, the experiment inspires curiosity-driven inquiry, inviting further exploration into the conductivity of diverse organic materials. From fruits to vegetables, natureoffers a myriad of substrates ripe for experimentation. By probing the electrical properties of these materials, scientists unlock insights into their chemical composition, paving the way for innovative applications in fields ranging from agriculture to materials science.Conclusion:In conclusion, the potato chopstick experiment epitomizes the beauty of scientific inquiry and discovery. Through a simple arrangement of everyday materials, it illuminates fundamental principles of conductivity, circuitry, and energy conversion. Beyond its educational value, the experiment ignites a spirit of curiosity, prompting us to explore the wonders concealed within the ordinary. As we delve deeper into the mysteries of the potato and chopsticks, we embark on a journey of discovery that transcends the confines of the laboratory, enriching our understanding of the natural world and inspiring innovations that shape our future.。
树能防止水土流失和减少土地变成沙漠的英语
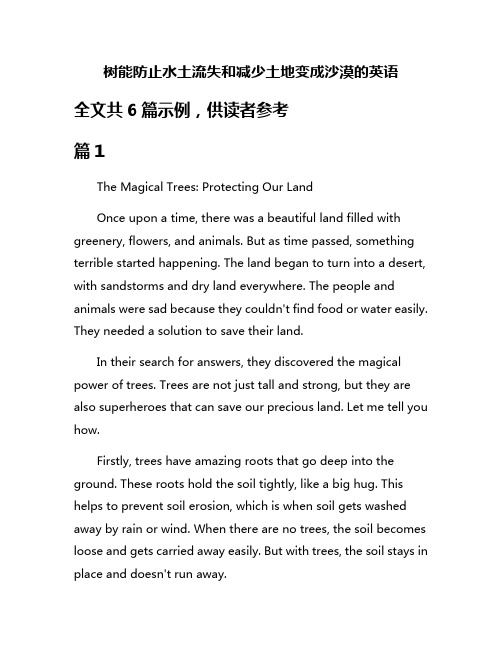
树能防止水土流失和减少土地变成沙漠的英语全文共6篇示例,供读者参考篇1The Magical Trees: Protecting Our LandOnce upon a time, there was a beautiful land filled with greenery, flowers, and animals. But as time passed, something terrible started happening. The land began to turn into a desert, with sandstorms and dry land everywhere. The people and animals were sad because they couldn't find food or water easily. They needed a solution to save their land.In their search for answers, they discovered the magical power of trees. Trees are not just tall and strong, but they are also superheroes that can save our precious land. Let me tell you how.Firstly, trees have amazing roots that go deep into the ground. These roots hold the soil tightly, like a big hug. This helps to prevent soil erosion, which is when soil gets washed away by rain or wind. When there are no trees, the soil becomes loose and gets carried away easily. But with trees, the soil stays in place and doesn't run away.Secondly, trees are like giant sponges. When it rains, they soak up the water and store it in their roots, trunks, and leaves. This is called "absorption." By doing this, trees help in reducing floods. Imagine if there were no trees, the rainwater would just flow on the ground, causing floods and washing away everything in its path. Trees save us from this disaster by absorbing the extra water.Another incredible thing that trees do is called "transpiration." It's like trees are always breathing out. They release water vapor through their leaves, just like how we exhale. This process helps to cool down the air and make it fresh. It's like trees have their very own air conditioning system! When the air is cool and fresh, it helps the clouds to form and bring rain. So, trees are like rainmakers too!Trees also provide shade, which means they create cool areas under their branches. This shade helps to keep the land and the animals living there safe from the scorching sun. It's like having a natural umbrella that protects us from getting too hot.Moreover, trees are homes for many animals. Birds build their nests on branches, squirrels make their homes in tree trunks, and insects live in the leaves. When we cut down trees, we take away these homes from the animals, making them sad andhomeless. So, by protecting trees, we are also protecting these wonderful creatures.In conclusion, trees are the true heroes of our land. They prevent soil erosion, reduce floods, create rain, provide shade, and give homes to animals. Without trees, our land would turn into a dry desert, and we would lose our beautiful home. So, let's all join hands and plant more trees. Every tree we plant is a step closer to saving our land and making it green and vibrant once again.Remember, even as young students, we can make a big difference. So, let's be tree superheroes and protect our land together!Word count: 489 words篇2Trees: Guardians Against Soil Erosion and DesertificationHave you ever watched a heavy rainstorm and noticed the water rushing down the street or hillside, carrying mud and dirt along with it? That's called soil erosion, and it's a big problem for our planet. Soil erosion can wash away precious topsoil, makingit difficult for plants to grow and causing land to become dry and barren, like a desert.But did you know that trees can help prevent soil erosion and stop the spread of deserts? Let me explain how!Holding the Soil TogetherTrees have a fascinating root system that spreads deep into the ground, acting like a natural anchor. These roots intertwine and grip the soil tightly, preventing it from being washed away by heavy rains or strong winds. Imagine trying to pull a rope out of a knot – that's how tough it is for the soil to escape the grasp of tree roots!The leaves and branches of trees also play a crucial role in protecting the soil. When raindrops fall from the sky, they can hit the ground with a lot of force, causing the soil to splash and break apart. However, the leaves and branches of trees act like a natural umbrella, breaking the impact of the raindrops before they reach the ground. This helps keep the soil intact and prevents it from being carried away by water.Slowing Down the WindHave you ever noticed how it's much calmer and less windy inside a forest compared to an open field? That's because treesact as natural windbreakers, slowing down the wind and reducing its erosive power.Imagine a strong wind blowing across a barren landscape –it can easily pick up loose soil particles and carry them away, contributing to the formation of deserts. But when trees are present, their trunks, branches, and leaves create a barrier that disrupts the wind flow, significantly reducing its strength and ability to erode the soil.Improving Water AbsorptionTrees don't just protect the soil from erosion; they also help the ground absorb and retain water more effectively. The roots of trees create tiny channels and pockets in the soil, allowing water to seep in rather than running off the surface. This helps prevent water from carrying away precious topsoil.Additionally, the fallen leaves and branches from trees decompose over time, creating a rich layer of organic matter called humus. Humus acts like a sponge, soaking up water and slowly releasing it to the plants and trees around it. This helps keep the soil moist and prevents it from drying out, which is a major cause of desertification.Restoring Degraded LandsIn areas where soil erosion and desertification have already taken a toll, planting trees can be a powerful solution to restore the land. As trees grow, their roots and canopy gradually rebuild the soil structure, making it more resistant to erosion and better able to retain water.Imagine a once-fertile field that has been turned into a barren, dusty wasteland by years of poor land management practices. By planting trees and allowing them to establish themselves, the soil can slowly regain its fertility, and the area can be transformed from a desert-like landscape into a lush, green oasis.Trees: The Guardians of Our PlanetAs you can see, trees play a vital role in protecting our planet's soil and preventing the spread of deserts. They hold the soil together with their mighty roots, break the impact of heavy rains, slow down erosive winds, improve water absorption, and even restore degraded lands.So the next time you see a tree, remember the incredible work it's doing to keep our soil healthy and our landscapes green. Trees are true guardians of our planet, and we should cherish and protect them as they protect us.篇3Trees: The Guardians of Our LandHello, kids! Have you ever wondered how trees help keep our land healthy and prevent it from turning into a desert? Today, we're going to explore the amazing ways trees protect our soil and fight against desertification.What is Desertification?Desertification is a fancy word that means the process of fertile land turning into a desert. This can happen when soil loses its nutrients and becomes dry and sandy, making it difficult for plants to grow. Deserts are areas with very little rainfall, and they have sandy or rocky landscapes with hardly any vegetation.So, how do trees help prevent this from happening? Let me tell you!Holding the Soil TogetherTrees have deep roots that spread out underground, acting like anchors for the soil. These strong roots hold the soil particles together, preventing them from being blown away by wind or washed away by rain. Without trees, the soil can easily be carried away, leaving the land bare and vulnerable to erosion.Reducing the Force of RainWhen it rains, the raindrops can hit the ground with a lot of force, especially if there's no vegetation to cushion the impact. This force can dislodge soil particles and cause them to be washed away. But trees act like umbrellas, breaking the fall of raindrops with their branches and leaves. The leaves also slow down the flow of water, giving it more time to gently soak into the soil instead of running off and carrying away the precious topsoil.Improving Soil QualityTrees are true magicians when it comes to improving soil quality. Their fallen leaves and branches decompose, adding nutrients and organic matter to the soil. This makes the soil richer and better able to support plant growth. Trees also create shade, which helps keep the soil cool and moist, preventing it from drying out too quickly.Providing WindbreaksIn some areas, strong winds can be a major cause of soil erosion. Trees act as natural windbreaks, slowing down the wind and preventing it from carrying away the topsoil. By plantingrows of trees, farmers and gardeners can create effective barriers against erosion caused by wind.Attracting WildlifeTrees don't just protect the soil; they also provide homes for many animals, birds, and insects. These creatures, in turn, play important roles in the ecosystem, such as pollinating plants, controlling pests, and helping to spread seeds. A healthy ecosystem with a diverse range of plants and animals is less likely to experience desertification.Real-Life ExamplesNow, let's take a look at some real-life examples of how trees have helped prevent desertification:The Great Green WallIn Africa, an ambitious project called the Great Green Wall aims to plant a massive forest across the entire continent. This wall of trees will act as a barrier against the expanding Sahara Desert, protecting the land and communities from further desertification.The Dust BowlIn the 1930s, a series of severe dust storms hit the Great Plains region of the United States and Canada. These storms were caused by years of intensive farming that had stripped the land of its protective vegetation. Once the trees and grasses were gone, the wind could easily pick up the loose soil, creating massive dust clouds that buried farms and homes. After this environmental disaster, known as the Dust Bowl, efforts were made to plant trees and restore the land.China's Green Great WallIn China, a massive project called the Three-North Shelterbelt Program has been ongoing since the 1960s. The goal is to plant a vast belt of trees spanning over 4,800 kilometers (3,000 miles) to protect cities and farmland from the expanding Gobi Desert. By 2050, it is estimated that this "Green Great Wall" will cover an area of 100 million acres, helping to prevent further desertification and improve air quality.Trees are truly amazing, aren't they? They not only provide us with oxygen, shade, and beautiful scenery but also play a vital role in protecting our land from turning into a desert. By planting more trees and taking care of our existing forests, we can help ensure that our planet remains green and healthy for generations to come.So, the next time you see a tree, remember to thank it for all the hard work it does in keeping our soil rich and our land fertile. Together, we can help these incredible guardians of our land continue their important work.篇4Certainly! Here's an article of around 2000 words, written in English and aimed at elementary school students, explaining how trees can prevent soil erosion and desertification:Trees: The Green Guardians of Our LandHave you ever seen a desert? It's a vast, dry land with little to no vegetation, where the sand stretches as far as the eye can see. While deserts are fascinating, you wouldn't want your neighborhood or your favorite park to turn into one, right? That's where trees come in – they're like superheroes protecting our land from becoming a desert!What is Soil Erosion?Soil erosion is when the top layer of soil, called topsoil, gets washed or blown away. This can happen due to wind, rain, or running water. Imagine a heavy rainstorm hitting a bare patch ofland – the water would quickly wash away the loose soil, leaving behind a messy, muddy trail. That's soil erosion in action!Why is Soil Erosion Bad?Soil erosion is a big problem because it can lead to the land becoming dry, barren, and unsuitable for growing plants or crops. Without healthy soil, it's hard for vegetation to thrive, and the land can slowly turn into a desert-like environment. That's no good for farmers, animals, or anyone who enjoys green spaces and fresh produce!How Do Trees Help?Trees are like superheroes when it comes to preventing soil erosion and desertification (the process of land becoming a desert). Here's how they help:Root Power!Trees have extensive root systems that spread deep into the soil, acting like anchors. These roots hold the soil in place, preventing it from being washed or blown away. Imagine trying to pull a firmly rooted tree out of the ground – it's nearly impossible because those roots are holding on tight!Leaf LitterThe fallen leaves, twigs, and bark from trees create a protective layer on the ground called leaf litter. This natural mulch helps shield the soil from the direct impact of rain and wind, reducing erosion. It's like a cozy blanket for the soil!Rain CatchersThe leaves and branches of trees act like tiny umbrellas, catching raindrops before they hit the ground. This slows down the force of the rain, preventing it from dislodging and carrying away soil particles.Wind BreakersTrees can act as natural windbreaks, reducing the speed and intensity of wind gusts that could otherwise blow away precious topsoil. Their sturdy trunks and branches create a barrier against strong winds, protecting the land beyond them.Moisture ManagersTrees help regulate the moisture content in the soil. Their roots absorb water during rainy periods, preventing excessive runoff and erosion. During dry spells, trees release moisture into the soil through their leaves, keeping the ground from drying out and becoming vulnerable to wind erosion.Real-Life ExamplesNow, let's look at some real-life examples of how trees have helped prevent soil erosion and desertification:The Dust BowlIn the 1930s, a series of severe droughts and poor farming practices in the Great Plains region of the United States led to massive soil erosion, creating a phenomenon known as the Dust Bowl. Millions of acres of topsoil were swept away by strong winds, causing widespread damage to farmlands and contributing to the creation of vast, desert-like conditions. Planting more trees and implementing better soil conservation methods helped combat this environmental disaster.The Great Green WallIn Africa, an ambitious project called the Great Green Wall aims to plant a vast belt of trees across the continent, spanning over 8,000 kilometers. This "wall" of vegetation is designed to combat desertification and soil erosion in the Sahel region, which borders the Sahara Desert. By planting millions of trees, the project aims to stabilize the soil, improve agricultural conditions, and provide economic opportunities for local communities.Coastal ProtectionTrees play a crucial role in protecting coastal areas from erosion caused by strong winds, waves, and storms. Mangrove forests, for example, act as natural barriers against coastal erosion, shielding the land behind them from the forces of the ocean. Their intricate root systems help anchor the soil, preventing it from being washed away by tides and currents.So, the next time you see a tree, remember that it's not just a pretty plant – it's a powerful ally in the fight against soil erosion and desertification. By protecting and planting more trees, we can help keep our land green, fertile, and free from the threat of turning into a desert wasteland.Remember, every tree counts, and together, we can be the heroes our planet needs!篇5Trees are Amazing! They Stop Dirt from Going Away and Keep Our Land from Turning into a DesertHi everyone! Today I want to talk about how cool trees are. Did you know that trees are like superheroes for the ground we walk on? They protect the soil and stop it from washing or blowing away. And get this - they even help fight deserts from spreading! Isn't that awesome?Let me explain how trees do these fantastic things. First, let's talk about soil erosion. Soil erosion is when soil (that's the dirt that covers the ground) gets washed or blown away by wind and rain. This can happen when there's nothing holding the soil in place, like no plants or trees growing there.If soil keeps eroding away, soon there won't be any good soil left for plants to grow in. That's really bad because we need soil for our crops like wheat, corn, fruits and veggies. Without soil, we can't grow those yummy foods! Soil erosion can also pollute rivers and lakes when all that loose dirt gets washed into the water.That's where trees come in to save the day! Their awesome roots spread out under the ground and grip onto the soil tightly. This stops the soil from being washed or blown away so easily. The roots act like anchors or nets holding the soil in place. Trees are like security guards protecting our soil!Speaking of roots, did you know tree roots also absorb water from the soil? This helps prevent soil from being over-saturated with too much water during big rainstorms. Too much water can loosen soil and cause it to erode faster. But trees soak up that extra water with their roots, so the soil stays drier and more stable.The leaves and branches of trees are heroes too! When raindrops hit the tree's leafy canopy, the leaves break up the force of the falling raindrops. This is so cool - instead of heavy raindrops pounding into the soil, the rain gets dispersed into a gentle mist or trickles down the tree trunks. This soft watering doesn't dislodge soil particles as much.Trees also block wind with their trunks and branches. When strong winds blow over bare soil, they can pick up and sweep the loose soil away, which is called wind erosion. But trees act as windbreaks, reducing wind speed and turbulence near the ground. This prevents the wind from whipping soil particles into the air as easily.On top of stopping erosion, trees help fight deserts from spreading into new areas too! Deserts form when land becomes too dry for plants to grow. With no plants covering the soil, it can quickly blow or wash away, creating more desert conditions.But trees can break this vicious cycle! Trees draw moisture up from underground water sources through their root systems. Then they release a lot of that moisture into the air through their leaves in a process called transpiration. This increases humidity levels in the air around the trees.When the air has more moisture and humidity, it's easier for water to condense into dew or fogs that can hydrate plants and soil. More plants can then grow, which prevents more desertification from occurring. Scientists call this the"re-vegetation" effect of trees. Cool, right?Woah, I know this is a lot of information! Let me sum it up quickly:Tree roots anchor into soil, preventing it from eroding awayLeaves and branches block heavy rain and winds that can displace soilRoots absorb excess water so soil doesn't get over-saturated and erodedTrees release moisture into the air, increasing local humidity levelsHigher humidity allows dew and fogs to form, hydrating soil and enabling re-vegetationMore vegetation prevents desertification from spreadingSo in short, trees use their entire bodies as erosion-control machines and desert-fighters! They protect soil, enable moreplant growth, and keep dry areas from expanding into deserts. Trees really are superheroes for the environment!We should appreciate trees and make sure to plant more of them. Every tree helps bind soil in place and increase moisture in the air. With more trees covering the land, we can conserve our precious soil and stop deserts from taking over. Let's all betree-huggers and help these eco-warriors on their quest to keep our world lush and green!篇6Trees are Awesome for Stopping Soil Erosion and Deserts!Do you know what soil erosion is? It's when wind and rain wash or blow away the precious soil from the ground. Soil is super important because it's what helps plants and crops grow. Without good soil, it's really hard for things to grow! Soil erosion happens a lot in areas with not many trees or plants covering the ground.When there are trees with their roots digging into the soil, their roots act like little anchors holding the soil in place. Tree roots spread out under the ground and grip onto the soil tightly so the wind and rain can't wash or blow it all away. The leavesand branches on the trees also help block heavy rain from pounding down directly on the soil.In places with no trees or plant cover, the soil can get washed or blown away much more easily during storms. Over time, more and more of the nutrient-rich top soil gets lost, making it really difficult for anything to grow there. This process is called desertification when it turns fertile land into desert.Let me tell you about a true story of how trees stopped awful desertification in China. In the 1950s, a super bad drought hit the Ningxia region. With no rain for years, crops died and there was terrible famine. The wind whipped up the loose, dry soil into huge dust storms that buried homes and villages!The Chinese government came up with an amazing plan to stop the spreading desert. They paid local people to start planting tons of trees across the region. These tough tree species could survive the dry conditions once they got established. Over decades, millions of acres of new forest took root across the Ningxia region.Those forests did an incredible job holding the soil down with their roots so the land didn't turn to desert. The trees also helped bring more rainfall back to the area. Now animals havereturned, vegetation grows, and farming is possible again all thanks to those tough, protective trees!Another example is the tiny African country of Burkina Faso. Back in the 1970s and 80s, droughts and soil erosion turned a third of their country into desert. But farmers came up with a simple yet genius idea - they dug half-moon shaped trenches around trees and crops to catch rain water run-off.These half-moon trenches surrounding trees allowed rain water to sink into the soil slowly instead of forming erosive runoff. This easy technique combined with planting more trees brought massive areas of Burkina Faso back from the brink of total desertification. Today trees cover over 3 million more acres compared to the 1980s!Trees are like superheroes for protecting soil and stopping deserts from spreading. Their roots anchor down the soil while their leaves and branches shield soil from intense rain and wind erosion. Trees also help draw moisture into an area, allowing plants and crops to grow better.Countries all over the world are now planting more trees to fight soil erosion and reverse desertification. In China, there are over 66 billion new trees planted since the 1970s, creating huge new forests. That's over 20 trees for every single person in China!India also set a world record by planting an incredible 66 million trees in just 12 hours across the country.While planting trees is crucial, protecting the forests we already have is just as important. Every year an area of forests the size of Portugal is cut down across the planet. Losing those trees makes soil erosion and expanding deserts much worse in those areas.So next time you see a big, beautiful tree with its branches stretching up to the sky, remember how amazing it is! That tree's roots are hard at work holding the soil together while its leaves and branches take the beating from wind and rain. Trees do such an awesome job at keeping soil healthy and preventing deserts from taking over.I hope this article showed you how incredibly important trees are for stopping soil erosion and desertification. The more we can plant trees and protect existing forests, the better we can make sure we have enough fertile soil to grow all the crops we need. Trees are like real-life super heroes for the environment!。
进出口专业英语词汇(L1)
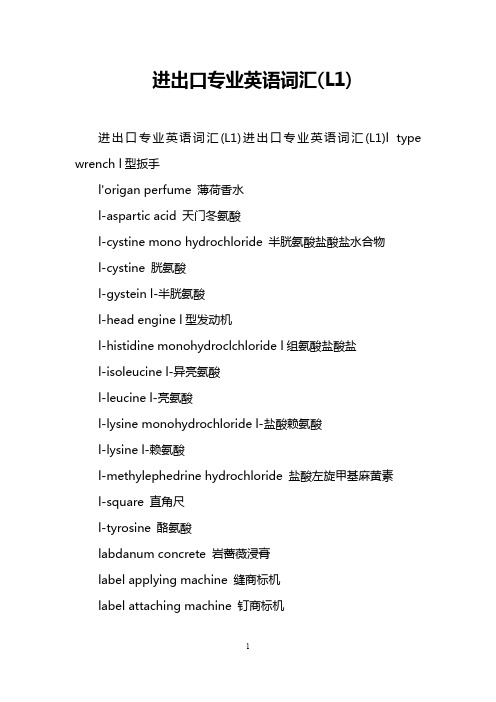
进出口专业英语词汇(L1)进出口专业英语词汇(L1)进出口专业英语词汇(L1)l type wrench l型扳手l'origan perfume 薄荷香水l-aspartic acid 天门冬氨酸l-cystine mono hydrochloride 半胱氨酸盐酸盐水合物l-cystine 胱氨酸l-gystein l-半胱氨酸l-head engine l型发动机l-histidine monohydroclchloride l组氨酸盐酸盐l-isoleucine l-异亮氨酸l-leucine l-亮氨酸l-lysine monohydrochloride l-盐酸赖氨酸l-lysine l-赖氨酸l-methylephedrine hydrochloride 盐酸左旋甲基麻黄素l-square 直角尺l-tyrosine 酪氨酸labdanum concrete 岩蔷薇浸膏label applying machine 缝商标机label attaching machine 钉商标机label gluing machine 粘标签机label gummer 商标上胶机label making machine 制标签机label marking machine 打商标机label paper 商标纸label printing machine 商标印刷机label remover 商标清除机label silk 商标缎label writer 标签印字器label-cutting press 标签裁压机label-sewing machine 缝标签机labeling machine 贴标签机labelled compound 标记化合物labeller 贴标签机labetalol 拉贝洛尔labile oscillator 易变振荡器labilite 津锰混剂labor axe 劳动斧laboratory apparatus 实验室器械laboratory appliance 实验室用具laboratory apron 实验室围裙laboratory centrifuge 实验室用离心机laboratory clothing 实验室工作服laboratory drying cylinder 实验室小烘筒laboratory electric stove 实验室电炉laboratory glassware 实验室玻璃仪器laboratory instrument and apparatus 实验室仪器与器械laboratory instrument 实验室仪器laboratory interferometer 实验室用干涉仪laboratory jigger 小染缸laboratory lamp 实验室用灯laboratory lathe 小打磨机laboratory mangle 小轧车laboratory microscope 实验室用显微镜laboratory mill 小磨机laboratory oscillator 实验室振荡器laboratory pliers 技工钳laboratory porcelain 实验室用瓷器laboratory printing machine 小印花机laboratory reagent 实验室试剂laboratory sifter 震动筛分机laboratory steamer 小蒸箱laboratory steaming chest 小蒸箱laboratory stirrer 实验室搅拌器laboratory thermometer 实验室温度计laboratory tray 实验室料盘laboratory vacuum pump 实验室用真空泵laboratory-size reactor 实验室型反应器labour axe head 劳动斧头labour protection appliance 劳保用品labour safety product 劳保产品labradorite 拉长石laburnham 莱本汉呢laburnum 莱伯南斜纹薄呢labyrinth box 迷宫密封箱labyrinth loudspeaker 迷宫式扬声器labyrinth oil retainer 迷宫式集油器labyrinth piston compressor 迷宫式活塞压缩机labyrinth piston 迷宫式活塞labyrinth valve 迷宫阀labyrinth-grease seal 迷宫式润滑脂密封器lac color 紫胶红色素lac resin 虫胶树脂lac varnish 虫胶清漆lac 虫胶lacarda 拉卡达牌手表lacasse machine 拉卡斯提花机laccaic acid 虫胶酸lacceroic acid 虫漆醋酸laccol 虫漆酚lace and band crochet machine 花边与带类钩编机lace and tiny tuck trim blouse 通纱细褶裥上衣lace and trimming machine 花边织机lace bark 网状韧皮纤维lace beaming machine 花边整经机lace bedspread 花边床罩lace bodice slip 胸背部通花衬裙lace boots 系带长统靴lace cloth 累丝纱lace cord 棉线绳lace curtain 花边窗帘lace doily 通花碟巾lace doubleknit 透孔双面针织物lace for edging 花边lace frame 花边织机lace furnishing machine 装饰花边机lace gloves 网眼手套lace hose 网眼袜lace machine 花边织机lace making machine 制花边机lace paper 花边纸lace print plastic drapery 印通花状塑胶帘lace ruffled yoke bed jacket 荷叶裥花边肩轭式床上短外衫lace silk 花边丝线lace stripe cloth 提花充纱罗条子布lace stripes 纱罗条纹织物lace table cloth 网眼花边台布lace trim bib 花边围涎lace trim dress 花边镶饰女孩外衫lace trimmed slip 镶花边套裙lace trimming 小花边lace yarn 花边纱线lace yoke frock 花边肩轭长外衣lace 花边lace-collared blouse 花边领上衣lace-curtain machine 花边窗帘织机lace-up snow boots 系鞋带踏雪靴laced corset 穿绳紧身褡laced paper cutter 花边纸刀laced shoes 缚带鞋laceless volley-ball 无线缝排球lacerating machine 拉力试验机lachka 拷花绸lachrymal dilator 泪腺扩张器lachrymal probe 泪腺探针lacing machine for jacquard cards 提花纹板串连机lacis 方网眼花边lacmus paper 石蕊试纸lacorda 拉可达牌手表lacovo biscuit 乐口福饼干lacovo 乐口福lacquer base 硝基漆基料lacquer basket 涂漆蓝子lacquer box 漆盒lacquer cable 漆包线lacquer chopsticks 漆筷lacquer enamel 珐琅lacquer film capacitor 喷漆薄膜电容器lacquer for floor 地板漆lacquer for pencil 铅笔漆lacquer furniture 喷漆家具lacquer painting 漆画lacquer picture 磨漆画lacquer prints 漆印花布lacquer screen inlaid carved jade 嵌老山玉屏风lacquer screen inlaid with carved ivory 嵌象牙屏风lacquer screen inlaid with carved mother-of-pearl 嵌螺钿屏风lacquer screen 喷漆屏风lacquer thinner 香蕉水lacquer tray inlaid with silver and mother-of-pearl 银丝螺钿漆托盘lacquer tray 漆盘lacquer varnish 喷涂清漆lacquer wall hanging 漆挂屏lacquer wax 漆蜡lacquer wood furniture 漆木家具lacquer 漆lacquer-removing preparation 除漆制剂lacquer-ware 漆器lacquered basket 漆篮lacquered cable 漆包电缆lacquered cotton hat 纱布喷漆帽lacquered fan handle 漆扇柄lacquered satin 橡皮缎lacquered wire 漆包线lacquering machine 喷漆机lacquerless metallized paper condenser 无漆金属化纸介电容器lacs d'amour 织花亚麻台布lactam 内酰胺lactase 乳糖酶lactasinum biofermin 乳酶生lactate 乳酸lactic acid amide 乳酸胺lactic acid 乳酸lactitolum monohydrate 一水乳梨醇lactobacillin tablet 乳酸菌素片lactobionic acid 乳糖醛酸lactochrome 核黄素lactodensimeter 乳比重计lactoflavin 核黄素lactogen 催乳激素lactometer 乳汁密度计lactone 内酯lactonic acid 内酯酸lactonitrile 乳腈lactoprene 聚酯橡胶lactoscope 乳脂计lactose 乳糖lactulose 乳果糖lactyl-lactic acid 乳酰乳酸lada 拉达牌汽车ladam cotton 拉大姆棉ladar 激光雷达ladder attenuator 梯形衰减器ladder dredger 链斗式挖泥机ladder fibre 梯形纤维ladder polysiloxane 梯形聚硅氧烷ladder tape for venetian blind 百叶窗带ladder trencher 多斗式挖沟机ladder truck 有梯卡车ladder type decoupling filter 梯型去耦滤波器ladder winch 梯式绞车ladder 梯子ladder-mounted drill 链斗式钻机ladder-type filter 梯型滤波器ladies men's jackets made out of sheep nappa suede 男女绵羊皮光面绒面茄克ladies men's leather trousers made out of nappa and suede 男女皮革光面绒面皮裤ladies cloth 薄型女式呢ladies dress 女式呢ladies ramie cotton skirt 女式麻棉裙ladies' men's jackets made out of goat nappa suede 男女羊皮光面绒面茄克ladies' apron 女式家用围裙ladies' cotton-padded jackets 女式棉茄克ladies' embroidered blouse 女式绣花上衣ladies' flat-egded printed handkerchief 女式平边印花手帕ladies' long coat 女大衣ladies' reversible cotton padded jacket 女式双面穿棉衣ladies' reversible silk wadding padded jacket 女装双面穿丝棉棉衣ladies' rubber rain boots in assorted colour 彩色女雨鞋ladies' scallopedged printed handkerchief 女式牙边印花手帕ladies' silk wadding padded jacket 女式丝棉棉衣ladies' wear 女装ladik rug 拉迪克地毯ladle car 桶车ladle with aluminium handle 铝柄汤勺ladle with wooden grip 木柄汤勺ladle 汤勺lado cotton 拉多棉lady amhurst pheasant feather duster with bamboo handle 竹柄铜鸡毛帚lady amhurst pheasant head and neck feather 铜鸡头项毛lady amhurst pheasant skin with tail 带尾铜鸡皮lady amhurst pheasant skin without tail 无尾铜鸡皮lady amhurst pheasant tail 铜鸡尾lady cracker 吨边炮lady shaver 女用电剃刀lady's bicycle 女式自行车lady's blouse 女衬衫lady's boots 女靴lady's cap 女帽lady's chinese gown 旗袍lady's clothing 女装lady's dress gloves 女式日用手套lady's embroidered blouse 女式绣花衬衫lady's embroidered jacket 女式绣花上衣lady's embroidered slippers with pvc sole 人造革底绣花女拖鞋lady's footwear 女鞋lady's frock 女连衫裙lady's garment 女服装lady's handbag 女用手提包lady's handkerchief 女用手帕lady's headwear 女帽lady's hosiery 女袜lady's house-shoes 女便鞋lady's jacket 女式茄克衫lady's leather coat 女式皮大衣lady's leather handbag 女用皮包lady's morning gown 女式晨衣lady's night gown 女式睡袍lady's pyjama 女式睡衣lady's quilted jacket vest 女式棉衣和棉背心lady's ready-to-wear 女式成衣lady's rocking chair 小摇椅lady's saddle 女用马鞍lady's shirt 女式衬衫lady's shoes cotton decorated with beads 棉串珠女鞋lady's shoes crocheted with cashmere 开士米女鞋lady's shoes 女鞋lady's slippers embroidery on cotton 棉绣花女拖鞋lady's slippers 女式拖鞋lady's style gloves 女式手套lady's textured stockings 女用网眼长袜lady's umbrella 女伞lady's underwear 女式内衣lady's wear 女装lady's wrist watch 女式手表ladybell root 南沙参ladys's topless type umbrella 女式折叠伞laevoglu cose 左旋葡萄糖laevorotatory oil 左旋油laffis 绢丝薄绸lag bolt 短螺栓lag compensator 滞后补偿器lag screw with square head 方头螺钉lager 窖藏啤酒lagging 圈架板lagonda 拉贡达牌汽车lagos cotton 拉格斯棉laguary cotton 拉瓜伊拉棉laharia 印度扎染布lahore chaddar shawl 拉合尔仿羊绒披巾lahore cloth 拉合尔羊绒花呢lahore 拉合尔羊绒花呢laid cigarette paper 条纹卷烟纸laid writing paper 条纹书写纸laid-in thread 衬垫纱线laine brodie 壁毯纬线laine de carmenie 伊朗山羊毛laine de terneaux 美利奴羊毛laine 羊毛laisot 优质低支亚麻帆布lake-grass floor mat 湖草地席lake-munia 色织花绸lake-wood 降香木lala 拉拉康铜lama 金属薄条lama-barchent 异纬双面绒布lamb ear plate 羔耳褥子lamb head plate 羔头褥子lamb head skin overcoat for child 羔头皮童大衣lamb leg plate 羔腿褥子lamb leg skin overcoat for child 羔腿皮童大衣lamb neck plate 羔脖褥子lamb pieces plate 羔皮材料褥子lamb skin hat for man 羔皮男帽lamb skin overcoat for child 羔皮童大衣lamb skin overcoat 羔皮大衣lamb skin parka for child 羔皮童皮猴lamb skin parka for lady 羔皮女皮猴lamb skin parka for man 羔皮男皮猴lamb skin parka 羔皮皮猴lamb skin plate 羔皮褥子lamb thigh plate 羔胯褥子lamb tongue 羊舌lamb's wool knitwear 羔羊毛毛衫lamb's wool yarn 短毛混纺纱lamb's wool 羔羊毛lamba shawl 兰巴披巾lamba 羔羊毛彩色披巾lambast 害草净lambert cotton 兰伯特棉lambertian reflector 朗伯反射器lambeth 兰贝思聚丙烯和聚乙烯单丝lambette 拉姆贝特高膨体腈纶吐波纱lamborghini 兰博尔吉尼牌汽车lambrol 联氟螨lambsdown 驼绒lambskin 劣质无烟煤lame yarn 金银丝花色线lamellar grating interferometer 层状光栅干涉仪lamina explosion-proof electric machine 窄隙防爆式电机laminac 拉明纳克聚酯树脂laminagraph 断层照相机laminar capacitor 片状电容器laminated adhesive 覆膜胶laminated armature conductor 叠片电枢导体laminated bag 涂膜袋laminated beam 叠层梁laminated board 层压板laminated brush switch 叠层刷型开关laminated brush 叠片电刷laminated chipboard 贴面碎料板laminated cloth 多层粘合布laminated coal 叠层煤laminated feeder 叠板式喂料机laminated ferrite memory 层压铁氧体存储器laminated film bag 层压薄膜袋laminated film 层压薄膜laminated glass partition wall 层压玻璃隔墙laminated glass 叠层玻璃laminated glassing paper 叠层玻璃纸laminated leaf-spring 多片式钢板弹簧laminated light-diffusing glass 夹层光漫射玻璃laminated material 层压材料laminated padlock 千层锁laminated paper 层压纸laminated phenolic paper 酚醛层压纸laminated plastic board picking stick 层压塑料板打梭棒laminated plastic board side lever 层压塑料板侧杆laminated plastics 层压塑料laminated plate lock 连片锁laminated plywood 胶合板laminated safety glass 层压安全玻璃laminated spring 叠板式弹簧laminated tarpaulin 层压帆布laminated thermosetting plastics 层压热固塑料laminated v-belt 活络三角带laminated wire glass 夹层嵌丝玻璃laminated wood board 细木工板laminated wood 层压板laminatedfibre band switch 胶板波段开关laminates 多层布laminating adhesive for foam plastics 泡沫塑料复合胶laminating press 层压压机laminating resin 层压树脂laminating roller 薄板轧辊laminating rolling mill 薄板轧机lamination detector 分层探测仪lamination synthetic leather 层压合成革laminator 折叠型压片机laminectomy retractor 单侧椎板拉钩lamita 拉米塔lammy 棉袄lamo 拉莫粘胶丝束lamont 拉蒙脱牌手表lamp lantern 灯类lamp annunciator 灯示呼唤器lamp base adapter 灯座连接器lamp bracket 灯架lamp brightness selector 灯光亮度调节器lamp bulb vacuum pump 灯泡真空抽气泵lamp bulb 灯泡lamp chimney 灯罩lamp condenser lens 光源聚光透镜lamp driver 灯驱动器lamp fittings 电灯组件lamp for optical pyrometer 光学高温计用灯炮lamp glass brush 玻璃灯罩刷lamp glass 灯罩lamp holder 灯座lamp house 灯罩lamp lens 车灯玻璃lamp ph-meter 灯式酸碱计lamp reflector 车灯反射镜lamp screen 灯罩lamp shade and reflector 灯罩及反射器lamp shade 灯罩lamp socket 灯座lamp stand with tiger and rock design 石虎灯座lamp stand 灯座lamp switch 灯开关lamp synchroscope 同步指示灯lamp system constant voltage generator 照明系统定压发电机lamp voltage regulator 白炽灯稳压器lamp wick 灯芯lamp 灯lampas du japon 经棱纹丝花缎lampas 彩花细锦缎lampasette 彩花斜纹绸lampblown glassware 灯工玻璃制品lamprecide 杀八目鳗剂lamsa 优质平布lamé金银锦缎lamélace 金银丝花边lamé拉梅金属薄膜丝lan-cer-amp 兰塞安普合金lan-mu-hsiang 兰木香lana fibre 拉纳木棉纤维lanabeta 拉纳贝塔蛋白质化粘胶纤维lanacron dye 拉纳克隆染料lanacryl 拉纳克里尔聚丙烯腈系纤维lanameter 羊毛纤维细度计lanatin 拉纳廷变性植物纤维lanatoside a 毛花甙alanatoside c 毛花甙clancashire boiler 卧式双火炉锅炉lancashire flannel 兰开夏色织白边法兰绒lancaster cloth 兰开斯特油布lance asiabell root 四叶参lance 喷管lance-type burner 喷枪式燃烧器lance-type sootblower 喷枪式吹灰器lancer ex 枪骑兵ex牌汽车lancer fiore 枪骑兵-菲奥尔牌汽车lancet 柳叶刀lanchi origin green soyabean 兰溪青豆lanchi origin yellow soyabean 兰溪黄豆lancia 兰恰牌汽车lancéembroidery 镶边刺绣land clearing blade 除荆机land conditioner 土地平整机land cruiser 陆地巡洋舰牌汽车land diesel engine 陆用柴油机land dredger 挖泥机land driver 陆上打桩机land engine 陆地发动机land grader 灌木清除机land leveller 平地机land levelling machine 平地机land otter fur 獭皮land receiver 地面接收机land roll 镇压器land roller 平地滚压器land rover 越野牌汽车land shaper 筑埂机land-barometer 陆用气压表landa 兰达牌手表landau 兰多牌汽车landau 双排座敞蓬轿车landaulet 单排座轿车landfill gas analyzer 填埋气体分析仪landia 兰地亚牌手表landing approach simulator 着陆进场模拟器landing approach trainer 着陆进场教练机landing beam transmitter 路道定位标发射机landing chassis 起落架landing craft 登陆舰landing light 着陆灯landing monitor 着陆监视器landing path monitor 着陆路径监控器landing ship 登陆艇landing skid 着地滑橇landing wire 提网钢索landing-site indicator 着陆点指示器landis chaser 切向螺纹梳刀landplane 陆上飞机landrin 混杀威landscape of plastics 塑料盆景landscape painting 风景画lane marker 车道划线机lanella fleece yarn 拉纳拉混纺纱lanella 拉纳拉棉毛法兰绒lanese 兰尼斯醋酯短纤维lanex 伏草隆langalloy 4r 兰氏4r耐蚀合金langalloy 5r 兰氏5r耐蚀合金langalloy 兰加洛伊高镍铸造合金langel 兰吉牌手表langet 梭结粗花边langevin echo sounding machine 兰格文回声测深仪langley 兰利牌汽车langouste 龙虾lanilla 硬挺整理精纺哔叽lanital 拉尼塔酪蛋白纤维lanital 人造羊毛lanitop 甲基地高辛lankart 兰卡特棉lannate 灭多虫lano wool 拉诺植物纤维lanoc cn 防蠹磺lanolin cream shampoo 羊毛脂洗头膏lanolin soap 羊毛脂皂lanolin 羊毛脂lanoline cream 润肤膏lanoline grease 纯羊毛脂lanolis 羊毛脂lanon 拉农聚酯纤维lansil 兰锡尔醋酯长丝lanslide 环隆合剂lanstan 壮棉丹lantern candle 灯笼蜡烛lantern coal 烛煤lantern lens 幻灯镜头lantern mantle 灯头纱罩lantern pinion 滚柱小齿轮lantern projector 幻灯放映机lantern shape powder enamel vase with flower and bird design 粉彩花鸟灯笼瓶lantern shape powder enamel vase with landscape design 粉彩山水灯笼瓶lantern slide projector 幻灯放映机lantern slide 幻灯片lantern 幻灯机lanthanite 镧酸盐lanthanum boride 硼化镧lanthanum carbide 碳化镧lanthanum chloride 氯化镧lanthanum concentrate rare earth chloride 富镧氯化稀土lanthanum fluoride 氟化镧lanthanum nitrate 硝酸镧lanthanum oxide 氧化镧lanthanum silicate 硅酸镧lanthanum titanate 钛酸镧lanthanum 镧lanthanum-enriched rare earth chloride 富镧氯化稀土lanusa 拉努扎lanvis tablet 兰快疗片laos 劳斯绉lap drag 叠板刮路机lap evenness tester 棉卷均匀度试验机lap former 条卷机lap joint flange 活套法兰lap joint stud 活套环圈lap roller 棉卷辊lap seam welder 搭焊机lap waste opener 皮辊花机lap welder 搭接焊机lap winding 叠绕组lap-robe 盖膝的毯子lap-welded pipe 搭焊管laparogastroscope 剖腹胃镜laparoscope 腹腔内窥镜laparotomy sponge 腹部手术巾lapeled vest 有领背心lapirium chloride 拉匹氯铵lapis lazuli carving 青金石雕刻品lapis lazuli 琉青金石lapkin 有钮扣眼的餐巾lapnis fibre 拉普尼斯韧皮纤维lappas 拉珀斯方平色条棉布lapper 研磨机lappet dotted swiss 浮纹点子薄细布lappet loom 浮纹织机lappet thread 刺绣线lappet yashimagh 浮纹面纱lappet 垂花机lapping agent 抛光剂lapping compound 研磨膏lapping fluid 磨料研磨液lapping liquid 研磨液lapping lubricant 研磨润滑剂lapping machine 卷板机lapping material for mono-crystalline silicon 单晶硅研磨材料lapping oil 研磨油lapping powder 研磨粉lapping surface plate 研磨平板lapping tool 研磨工具lapping wheel 研磨轮laprotomy sponge 纱布巾lapseam pyramid coat 角锥形叠缝大衣laray viscometer 莱雷粘度计larch 落叶松lard oil 猪油lard white ware 猪油白lard 猪油lardo 拉度牌手表large core storage 大容量磁心存储器large ac three-phase synchronous motor 大型交流三相同步电动机large air compressor 大型空气压缩机large and medium dc motor 大中型直流电动机large area photomultiplier 大面积光电倍增器管large arm caliper 大臂井径仪large black colored kidney bean 大黑花芸豆large bolus for activating channels and collaterals 大活络丸large capacitor checking instrument 大电容校验仪large capacity disc 大容量磁盘large capacity meter 大流量计large capacity refrigerated centrifuge 大容量冷却离心机large cassette tape recorder 大盒式磁带录音机large cherry 大樱桃large cherry-apple preserved 大海棠脯large cnc wire-cut edm machine 大型数控电火花线切割机床large coloured kidney bean 大花芸豆large conical gear bolt 大圆锥齿轮螺栓large conical gear 大圆锥齿轮large contour projector 大型轮廓投影仪large core fiber 粗芯光纤large core high numerical aperture fiber 大芯径大数值孔径光纤large core memory 大容量存储器large extension table 大号伸长台large festive fireworks 礼花弹large flat head slotted screw 大平槽头螺钉large gas engine 大型气体发动机large gear cutting machine 大型齿轮加工机床large gearring 大齿轮圈large green chillie in brine 盐渍大绿辣椒large induction motor 大型感应电机large inside micrometer calliper 大内径测微卡尺large matrix store 大容量矩阵存储器large mobile fire extinguisher 大型移动式灭火机large module gear 大模数齿轮large open lamp with stand 带灯座大口灯large optical reflector 大型反光镜large optical-cavity diode 大光腔二极管large optical-cavity laser diode 大光腔激光二极管large oval head nibbed bolt 大扁圆头带榫螺栓large oval head rivet 大扁圆头铆钉large oval head socket shank rivet 大扁圆头半空心铆钉large oval head square neck bolt 大扁圆头方颈螺栓large owl 大猫头鹰large pill 大药丸large power tube 大功率电子管large power relay 大功率继电器large power transistor 大功率晶体管large pressurized vessel 大型压力容器large purple coloured kidney bean 大紫花芸豆large red chilli in brine 盐渍大红辣椒large red fish milk chocolate bean 大红鱼牛奶朱古力豆large research microscope 大型研究用显微镜large saw 大锯large scale computer 大型计算机large scale integrated chip 大规模集成电路芯片large scale integrated circuit 大规模集成电路large scale 大秤large screen display 大屏幕显示器large screen projector 大屏幕投影机large screen radar indicator 大屏幕雷达指示器large screen television 大屏幕电视机large screen video projector 大型屏幕录像投影机large shaft sleeve 大轴套large size coconut fibre brush 大号椰子纤维刷large size folding wood dryer 大型折叠木晾衣架large size reverberatory furnace 大型反射炉large space telescope 大型空间望远镜large spun rayon square 人造丝大方巾large square bland continuous-casting machine 大方坯连铸机large sweet broad peas 大荷兰豆large tea spoon 大茶匙large tom-tom 爵士乐手鼓large toolmaker's microscope 大型工具制造工用显微镜large transfer press 重型连续压力机large transport airplane 大型运输机large twill 斜纹布large type dozer 大型推土机large type excavator 大型挖掘机large type drill 大型钻机large value capacitor 大容量电容器large volume data exchanger 大容量数据交换机large white kidney bean 大白芸豆large-bus 大型公共汽车large-capacity motorcycle 重型摩托车large-curtain projecting tv set 大屏幕投影电视large-duty loader 大型装载机large-headed atractylodes rhizome 白术large-headed atractylodes 白术large-size high frequency hydraulic vibration table 大型高频液压振动台large-size luxurious travelling bus 豪华旅游大客车large-size passenger bus 大客车large-size precision thread ring and plug gauge 大型精密螺纹环塞规large-sized diesinker 大型锻模仿型铣床large-sized generator rotor slotting machine 大型电机转子槽铣床large-sized long arm excavator 大型长臂挖掘机large-sized photographic microscope 大型照相显微镜large-type horizontal metallurgical microscope 大型卧式金相显微镜large-type passenger plane 大型客机large-type sinterer 大型烧结机large-type tractor 大型拖拉机large-width cloth cutter 宽幅布切布机laria cotton 拉里亚棉laristan 拉利斯顿地毯larsen potentiometer 拉森电位计larva of a silkworm with batrytis 僵蚕larvacide 氯化苦larvae exterminating preparation 灭幼虫制剂larvatrol 苏云金杆菌larvicide 杀幼虫剂laryngeal mirror 反光喉镜laryngeal polypus forceps 喉息肉钳laryngeal scissors 喉头剪子laryngograph 喉动描记器laryngoscope 喉镜laryngostroboscope 喉动态镜larynx atomizer 喉头喷雾器lasalocid 拉沙里菌素laser absolute gravity meter 激光绝对重力仪laser acupuncture instrument 激光针灸机laser acupuncture treatment instrument 激光针灸治疗仪laser alarm detector 激光报警探测器laser altimeter 激光测高计laser amplifier 激光放大器laser and plasma cutting machine 激光和等离子切割机laser ash density meter 激光粉尘浓度计laser beam coupler 激光束耦合器laser beam flying spot scanner 激光束飞点扫描器laser beam image reproducer 激光束图像重现器laser beam scanner 激光束扫描器laser bender 激光结扎器laser benioff strain seismometer 激光贝尼奥夫应变地震仪laser blood cell counter 激光血球计数器laser calorimeter 激光量热器laser camera 激光摄影机laser cavitation meter 激光气蚀计laser ceramics 激光陶瓷laser cloth inspector 激光验布器laser coelostat 激光定星镜laser collimator 激光准直仪laser computer 激光计算机laser connector 激光器连接器laser corrosion monitor 激光腐蚀监测仪laser cosmetic instrument 激光美容仪laser crystal 激光晶体laser cure apparatus 激光治疗机laser cutter 激光切割机laser cutting machine 激光切割机laser deflector 激光偏转器laser demodulator 激光解调器laser detection equipment 激光检测设备laser detector 激光探测器laser diode amplifier 激光二极管放大器laser diode coupler 激光二极管耦合器laser diode demodulator 激光二极管解调器laser diode detector 激光二极管检测器laser diode driver 激光二极管激励器laser diode tester 激光二极管测量仪laser diode 激光二极管laser direction finder 激光测向仪laser disc digital audio player 数字式激光盘唱机laser disc player 激光放像机laser doppler perfusion flowmeter 激光多普勒微循环血流仪laser doppler velocimeter 激光多普勒速度计laser driller 激光钻孔机laser drilling machine 激光钻孔机laser driver 激光器驱动器laser earthquake alarm 激光地震报警器laser emulsion storage 激光乳胶存储器laser energy meter 激光能量测量仪laser energy monitor 激光能量监控器laser face beautifier 激光美容仪laser facsimile apparatus 激光传真机laser fantasy 激光幻影器laser fault detector 激光探伤仪laser fibre coupler 激光纤维耦合器laser fibre 激光纤维laser flash lamp 激光闪光灯laser flash tube 激光闪光管laser flowmeter 激光流量计laser fluorimeter 激光荧光计laser for medical purpose 医用激光器laser frequency multiplier 激光倍频器laser fusion cutting machine 激光核聚变切割机laser generator 激光发生器laser glass 激光玻璃laser goggles 激光防护镜laser granulometry 激光粒度测定仪laser gravitometer 激光重力仪laser guided bomb 激光制导炸弹laser gun 激光枪laser gyro 激光罗经laser gyroscope 激光陀螺仪laser heat-treatment machine 激光热处理机laser holographic interferometer 激光全息干涉仪laser illuminator 激光施照器laser image recorder 激光图像记录器laser imaging radar 激光成像雷达laser intercept receiver 激光截获接收机laser interferometer 激光干涉仪laser intrusion detector 激光入侵探测器laser inverter 激光变换器laser irradiation detector 激光辐射探测器laser jaw recorder 激光颌牙活动记录器laser jet printer 激光喷射式打印机laser length measuring machine 激光线纹测长机laser level 激光水平仪laser liquid flowmeter 激光液体流量计laser listening device 激光侦听器laser locator 激光定位器laser marking machine 激光标记机laser material 激光材料laser measuring machine 激光测量机laser microcirculating blood flow meter 激光微循环血流计laser microprobe mass analyzer 激光微探针质谱分析仪laser microprobe 激光微探针laser microscope 激光显微镜laser microspectral analyzer 激光显微光谱分析仪laser mirror 激光镜laser modulator 激光调制器laser monitor for pollution 激光测污仪laser monochromator 激光单色仪laser ophthalmic therapy apparatus 激光眼科治疗机laser optical coating 激光光学涂料laser optical demonstration instrument 激光光学演示仪laser oscillator 激光振荡器laser particle monitor 激光粒子监控器laser photo coaguator 激光光凝固器laser photocomposing machine 激光照排机laser phototypesetting film 激光照相排字软片laser plane interferometer 激光平面干涉仪laser platemaker 激光制版机laser plotter 激光绘迹仪laser points therapeutic apparatus 激光穴位治疗仪laser power meter 激光功率计laser preamplifier 激光前置放大器laser precision liquid level sensor 激光精密液位计laser pressure gauge 激光压力计laser printer 激光打印机laser pump 激光泵laser radar 激光雷达laser radiation detector unit 激光辐射探测器laser raman spectrometer 激光拉曼光谱仪laser range finder 激光测距仪laser ranger 激光测距仪laser ray nondestructive tire tester 激光轮胎无损检验仪laser ray tube 激光射线管laser receiver 激光接收机laser record 激光唱片laser recorder 激光记录器laser repeater 激光中继器laser retina photocoagulator 激光视网膜凝固器laser retinal mtf tester 激光视网膜mtf测定仪laser satellite tracking system 激光卫星跟踪仪laser scanned acoustic camera 激光扫描声学摄影机laser scanning analyser 激光扫描分析器laser schieren apparatus 激光纹影仪laser seeker 激光寻的器laser semiconductor 激光半导体laser sensor 激光传感器laser sonar 激光声纳laser spot tracker 激光点跟踪器laser stamping paper 激光打印纸laser strainmeter 激光应变计laser streak velocimeer 激光条纹测速仪laser submarine range finder 激光水下测距仪laser surface treatment machine 激光表面处理机laser surgical apparatus for ophthalmological treatment 激光眼科手术仪laser sword 激光剑laser theodolite 激光经纬仪laser therapeutic and cosmetic system 激光治疗美容机laser therapeutic instrument 激光治疗仪laser therapy and accupuncture instrument 激光理疗针灸仪laser therapy apparatus 激光治疗机laser toy gun 激光玩具枪laser tracker 激光跟踪器laser transceiver 激光收发信机laser transmitting set 激光发射机laser treater 激光治疗仪laser triggered switch 激光触发开关laser trimmer 激光修整机laser tripler monochrometer 激光三联单色光度计laser tube 激光管laser vector velocimeter 激光矢量速度计laser velocimeter 激光速度计laser vision tester 激光视力仪laser warning receiver 激光报警接收器laser wattmeter 激光功率计laser welder 激光焊机laser writer 激光印字机laser 激光器laser 莱塞牌汽车laser-beam plotter 激光绘图仪laser-beam-expanding telescope 激光扩束望远镜laser-doppler anemometer 激光-多普勒风速计laser-light protective goggles 激光防护镜laser-probe emission spectrometer 激光探头发射光谱仪laser-shoot imitater 激光射击模拟器laser-to-fiber positioner 激光器至光纤定位器lasercomp 激光照相排字机lash type inertia vibrating shakeout 冲击式惯性振动落砂机lashed fiber optic cable 缠挂式光缆lashed pile velveteen 扣紧纬平绒lasing fibre 激光纤维lasing protective filter 激光防护滤光片lasiosphaera seu calvatia 马勃laska skin cape for lady 香鼠皮女披肩laska skin plate 香鼠皮褥子laska skin scarf for lady 香鼠皮女围巾laska skin stole for lady 香鼠皮女披肩laska skin 香鼠皮lasso 草不绿lasso+bromex 草磷混剂lasso+maloran 草溴隆混剂lasso+sencor 草不津混剂last copy machine 鞋楦仿形机last for shoe 鞋楦last 鞋楦last-molding machine 鞋楦头加工机lastet capsule 拉司太特胶囊lasting cementing machine 帮角刷胶机lasting yarn 强力纱lasting 厚实斜纹织物lastochka 海燕牌手表lastrine 元色塔夫绸lasurite 天青石latamoxef 拉他头孢钠latch block 闭止块latch handle 闭止把latch needle machine 舌针针织机latch needle warp knitting machine 舌针经编机latch plate 插销latch rod 闭止杆latch 门闩锁latch-lock 弹子门锁latching relay 自锁继电器late pink 剪秋罗late potential analyser 晚电位分析仪late spring tea 三春茶latent crimp staple 潜卷缩性短纤维latent crimp yarn 潜卷缩性纱线latex additive 乳胶添加剂latex back full-cut rug 胶背全片地毯latex backed carpet 乳胶涂底地毯latex balloon 乳胶气球latex catheter 乳胶导管latex cement 乳胶水泥latex drainage tubing 乳胶引流管latex examination gloves 乳胶检查手套latex finger cot 乳胶医用指套latex foam core mattress 液泡树胶垫latex foam pillow 液泡树胶枕latex gloves 乳胶手套latex house paint-matt 乳胶无光建筑涂料latex household gloves 乳胶家用手套latex industrial gloves 乳胶工业手套latex mixer 胶浆搅拌机latex paint 乳胶漆latex products 乳胶制品latex prophylactic 乳胶避孕套latex rubber bag 乳胶袋latex rubber 乳胶橡胶latex separator 胶液分离机latex suction tube 乳胶吸管latex surgical gloves 乳胶手术手套latex tube for blood transfusion 乳胶输血管latex tube for stethoscope 听诊器用乳胶管latex 乳胶latex-back full-cut woollen rug 胶背全片纯毛地毯latex-glueing machine 乳胶粘合机latexometer 乳胶比重计lath nail 向耙钉lath 板条lathe bit 车刀lathe carrier 车床鸡心夹头lathe centre 车床顶尖lathe chuck 车床夹盘lathe cramp 大轧头lathe cutter 车床切削刀具lathe dog 鸡心夹头lathe face plate 花盘lathe for copper roller 车花筒机lathe for wood-work 木工车床lathe frame 车床架lathe spanner 车床螺丝扳手lathe tool 车刀lathe tool-setting microscope 车床对刀显微镜lathe 车床lathe-drill 卧式钻床lather shaving cream 泡沫刮须膏lathing hatchet 锤斧laticifer 乳计器laticiferous vessel 乳胶容器laticometer 乳胶比重计latigo leather 矾鞣革lattice beam 格构梁lattice brick 格子砖lattice filter 网格滤波器lattice girder 格构梁lattice tower 格子塔lattice type quantity equipment 格式定量器latvia 拉脱维亚牌汽车laudexium metilsulfate 劳地铵甲硫酸盐laughing buddha 笑佛launch vehicle 运载火箭launch 汽艇。
小学上册第9次英语第五单元测验卷
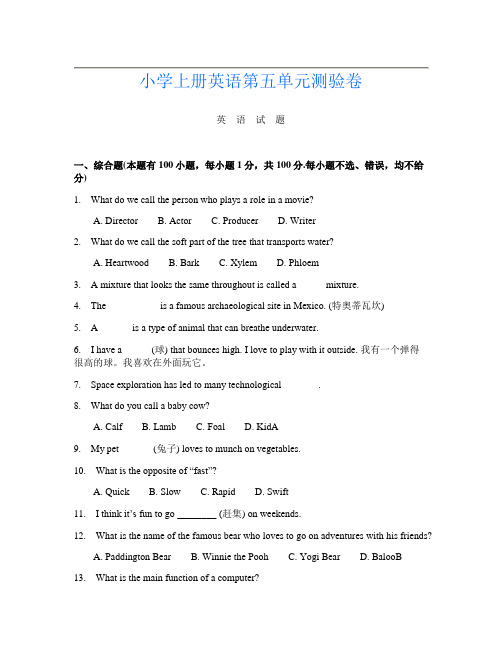
小学上册英语第五单元测验卷英语试题一、综合题(本题有100小题,每小题1分,共100分.每小题不选、错误,均不给分)1.What do we call the person who plays a role in a movie?A. DirectorB. ActorC. ProducerD. Writer2.What do we call the soft part of the tree that transports water?A. HeartwoodB. BarkC. XylemD. Phloem3. A mixture that looks the same throughout is called a _____ mixture.4.The __________ is a famous archaeological site in Mexico. (特奥蒂瓦坎)5. A ______ is a type of animal that can breathe underwater.6.I have a _____ (球) that bounces high. I love to play with it outside. 我有一个弹得很高的球。
我喜欢在外面玩它。
7.Space exploration has led to many technological _______.8.What do you call a baby cow?A. CalfB. LambC. FoalD. KidA9.My pet ______ (兔子) loves to munch on vegetables.10.What is the opposite of “fast”?A. QuickB. SlowC. RapidD. Swift11.I think it’s fun to go ________ (赶集) on weekends.12.What is the name of the famous bear who loves to go on adventures with his friends?A. Paddington BearB. Winnie the PoohC. Yogi BearD. BalooB13.What is the main function of a computer?A. To cook foodB. To process informationC. To cleanD. To provide entertainmentB14.n Wall came down in ________ (1989). The Berl15.I like to ___ (collect) stamps from different countries.16.What is the name of the holiday celebrated on October 31st?A. ThanksgivingB. ChristmasC. HalloweenD. New YearC17.Metals are usually good __________ of heat and electricity.18.How many zeros are in one hundred?A. 1B. 2C. 3D. 4B19.The playground is ________.20.The ____ is a wise animal often found in forests.21. A covalent bond is formed when two atoms ________ electrons.22.Which instrument has strings and is played with a bow?A. GuitarB. ViolinC. PianoD. Flute23.What do we call a young cat?A. PupB. KittenC. CalfD. ChickB24. A ______ is a type of substance that can change its state.25.The ____ has a bright blue color and is often found near water.26.Parrots can ______ human speech.27.My _____ (哥哥) helps me with my homework.28.My sister is my best _______ who is always ready to help me.29.In my free time, I like to play _______ (游戏). It helps me relax and have fun.30.The flowers are ___. (blossoming)31.The Earth's crust is made up of both igneous and ______ rocks.32.What do we call the act of cleaning dishes?A. WashingB. ScrubbingC. DryingD. Rinsing33. A ____ has long whiskers and enjoys scurrying about.34.What sound does a cow make?A. MeowB. MooC. BarkD. QuackB35.Cosmic rays are high-energy particles that travel through ______.36.We are going to ________ a trip.37.The _______ can thrive in challenging conditions.38.The ______ (绿色植物) purify the air.39.My ________ (玩具名称) is a great partner for learning.40.My uncle is a ______. He travels a lot for work.41.What is the name of the famous character who loves to eat lasagna?A. GarfieldB. OdieC. JonD. Pooky42.What is the name of the toy that you can build with blocks?A. PuzzleB. LegoC. DollD. CarB43.What do we call a group of owls?A. ParliamentB. FlockC. GaggleD. SchoolA Parliament44.Hamsters like to run on a ________________ (轮子).45. A ____(green certification) recognizes sustainable practices.46.The ________ was a renowned leader in the civil rights movement.47. A __________ is a natural opening in the ground where hot gas and steam can escape.48.The storm is ______ outside. (raging)49.The chameleon can hide in the _________. (树叶)50.The ________ is a friend to all who visit.51.The Great Lakes are located in __________.52.An oxidizing agent is a substance that ________ electrons.53.The ______ works at the library.54.My cousin is always . (我的表兄弟/表姐妹总是。
化学耕作与有机耕作相比较英语作文
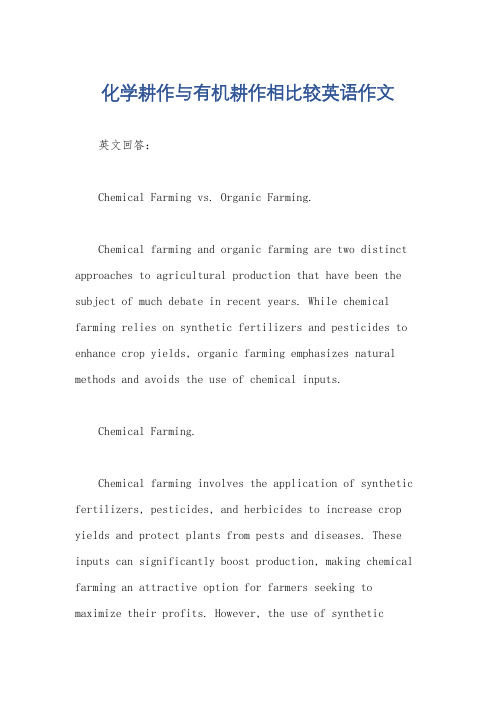
化学耕作与有机耕作相比较英语作文英文回答:Chemical Farming vs. Organic Farming.Chemical farming and organic farming are two distinct approaches to agricultural production that have been the subject of much debate in recent years. While chemical farming relies on synthetic fertilizers and pesticides to enhance crop yields, organic farming emphasizes natural methods and avoids the use of chemical inputs.Chemical Farming.Chemical farming involves the application of synthetic fertilizers, pesticides, and herbicides to increase crop yields and protect plants from pests and diseases. These inputs can significantly boost production, making chemical farming an attractive option for farmers seeking to maximize their profits. However, the use of syntheticchemicals in agriculture has raised concerns about environmental and health impacts.Environmental Impacts: Chemical fertilizers can lead to water pollution through runoff and leaching, contributingto eutrophication and algal blooms. Pesticides and herbicides can harm beneficial insects, pollinators, and other wildlife. They can also accumulate in the soil and groundwater, posing long-term risks to ecosystems.Health Impacts: Exposure to synthetic pesticides has been linked to a range of health problems, including cancer, reproductive disorders, and developmental issues. Residuesof these chemicals can also be found in food, potentially posing health risks to consumers.Organic Farming.Organic farming is a system of agricultural production that emphasizes the use of natural inputs and practices. It seeks to promote soil health, reduce environmental impacts, and enhance the nutritional value of food. Organic farmersrely on crop rotation, cover crops, and natural pest management techniques to maintain healthy and productive ecosystems.Benefits of Organic Farming.Organic farming offers several advantages over chemical farming, including:Improved soil health: Organic practices build soil biodiversity and organic matter, leading to improved soil structure, water retention, and nutrient availability.Reduced environmental impacts: Organic farming minimizes pollution and protects biodiversity by avoiding synthetic chemicals.Enhanced nutritional value: Studies have shown that organic produce contains higher levels of antioxidants, vitamins, and minerals compared to conventionally grown foods.Consumer health: Organic foods are generally free from synthetic pesticide residues, reducing potential healthrisks for consumers.Challenges of Organic Farming.Organic farming also presents some challenges, including:Lower yields: Organic farming typically produces lower yields than chemical farming, making it less profitable for farmers in some cases.Higher labor costs: Organic farming requires morelabor-intensive practices, such as weeding and pest control, which can increase production costs.Availability: Organic produce may be more expensiveand less widely available than conventionally grown foods.Conclusion.Chemical farming and organic farming represent distinct approaches to agricultural production with different environmental, health, and economic implications. While chemical farming offers higher yields and lower production costs, it raises concerns about environmental pollution and health risks. Organic farming, on the other hand, emphasizes sustainability, environmental protection, and nutritional value. Ultimately, the choice between chemical and organic farming depends on individual values,priorities, and market demands.中文回答:化学耕作与有机耕作。
颗粒有机碳和矿物结合有机碳测定方法
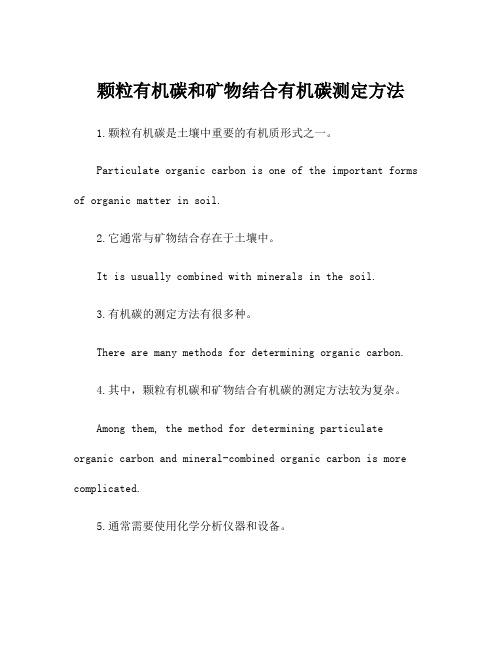
颗粒有机碳和矿物结合有机碳测定方法1.颗粒有机碳是土壤中重要的有机质形式之一。
Particulate organic carbon is one of the important forms of organic matter in soil.2.它通常与矿物结合存在于土壤中。
It is usually combined with minerals in the soil.3.有机碳的测定方法有很多种。
There are many methods for determining organic carbon.4.其中,颗粒有机碳和矿物结合有机碳的测定方法较为复杂。
Among them, the method for determining particulate organic carbon and mineral-combined organic carbon is more complicated.5.通常需要使用化学分析仪器和设备。
Chemical analysis instruments and equipment are usually required.6.这些仪器和设备可以帮助分离和测定颗粒有机碳和矿物结合有机碳。
These instruments and equipment can help separate and determine particulate organic carbon and mineral-combined organic carbon.7.在土壤样品中,颗粒有机碳和矿物结合有机碳的含量通常不同。
The content of particulate organic carbon and mineral-combined organic carbon in soil samples is usually different.8.有时候需要分别测定它们的含量。
实验室污水检测流程及标准
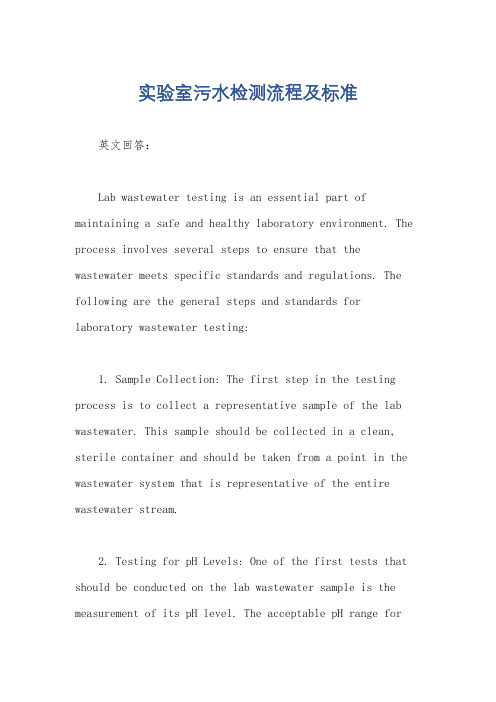
实验室污水检测流程及标准英文回答:Lab wastewater testing is an essential part of maintaining a safe and healthy laboratory environment. The process involves several steps to ensure that the wastewater meets specific standards and regulations. The following are the general steps and standards for laboratory wastewater testing:1. Sample Collection: The first step in the testing process is to collect a representative sample of the lab wastewater. This sample should be collected in a clean, sterile container and should be taken from a point in the wastewater system that is representative of the entire wastewater stream.2. Testing for pH Levels: One of the first tests that should be conducted on the lab wastewater sample is the measurement of its pH level. The acceptable pH range forlab wastewater is typically between 6 and 9. Any values outside of this range may indicate the presence of contaminants or pollutants.3. Chemical Analysis: Various chemical tests should be conducted to determine the presence of specific contaminants in the lab wastewater. This may include tests for heavy metals, organic compounds, and other pollutants that could be harmful to the environment.4. Biological Oxygen Demand (BOD) Testing: BOD testing is used to measure the amount of dissolved oxygen that is consumed by microorganisms in the lab wastewater. This test helps to assess the level of organic matter present in the wastewater and the potential impact on the receiving water bodies.5. Total Suspended Solids (TSS) Analysis: TSS testing is used to measure the amount of solid particles that are suspended in the lab wastewater. High levels of TSS can indicate poor water quality and may require additional treatment before the wastewater can be discharged.6. Compliance with Regulations: The results of the lab wastewater testing should be compared to local, state, and federal regulations to ensure that the wastewater meets all necessary standards for discharge.中文回答:实验室污水检测是保持实验室环境安全和健康的重要组成部分。
三水软铝石氧化铝生产过程中草酸盐脱除工艺影响因素研究

世界有色金属 2023年 7月上226三水软铝石氧化铝生产过程中草酸盐脱除工艺影响因素研究苌建新(广西华昇新材料有限公司,广西 防城港 538003)摘 要:我国的铝土矿资源日趋枯竭,使用富含有机物的三水软铝石生产氧化铝是大势所趋。
进口的铝土矿由于矿源的影响,有机物的含量较高一般在0.2%~0.4%之间,所以,进口矿源所带来的草酸盐浓度增加尤其值得重视。
草酸盐的脱除受多种因素的影响,其中主要影响因素有草酸盐的溶解度、草酸盐脱除不同的工艺方法以及草酸盐的苛化率三个方面。
本文将针对这些影响因素的作用规律进行分析,以达到有效地引导工艺优化,提高草酸盐的脱除效率的目的,从而为寻求进一步的草酸盐脱除处理方法奠定基础。
关键词:金属材料;三水软铝石;草酸盐脱除中图分类号:X758 文献标识码:A 文章编号:1002-5065(2023)13-0226-3Study on Influencing Factors of Oxalate Removal Process in Anhydrite Alumina ProductionCHANG Jian-xin(Guangxi Huasheng New Materials Co.,Ltd.,Fangchenggang 538003,china)Abstract: China 's bauxite resources are drying up, the use of organic-rich three water soft alumina production is the general trend. Imported bauxite due to the impact of mineral sources, the higher the content of organic matter is generally between 0.2%~0.4 %, so the import source brought about by the increase in oxalate concentration is particularly worthy of attention. The removal of oxalate is affected by many factors, among which the main influencing factors are the solubility of oxalate, the different process methods of oxalate removal and the causticization rate of oxalate. In this paper, the action rules of these influencing factors will be analyzed to effectively guide the process optimization and improve the removal efficiency of oxalate, so as to lay a foundation for further oxalate removal treatment. Keywords: metal materials ; alumina trihydrate ; oxalate removal收稿日期:2023-04作者简介:苌建新,男,生于1982年,汉族,河南郑州人,本科,研究方向:有机物脱除。
- 1、下载文档前请自行甄别文档内容的完整性,平台不提供额外的编辑、内容补充、找答案等附加服务。
- 2、"仅部分预览"的文档,不可在线预览部分如存在完整性等问题,可反馈申请退款(可完整预览的文档不适用该条件!)。
- 3、如文档侵犯您的权益,请联系客服反馈,我们会尽快为您处理(人工客服工作时间:9:00-18:30)。
Laboratory drying of organic-matter rich soils:Phosphorus solubilityeffects,influence of soil characteristics,and consequencesfor environmental interpretationDavid Styles a,c,⁎,Catherine Coxon b,caDepartment of Botany,Trinity College,University of Dublin,Dublin 2,Ireland bDepartment of Geology,Trinity College,University of Dublin,Dublin 2,Ireland cCentre for the Environment,Trinity College,University of Dublin,Dublin 2,IrelandReceived 31August 2005;received in revised form 3March 2006;accepted 10March 2006Available online 21April 2006AbstractAgronomic soil test P (STP)and soil type data may be used to predict diffuse soluble P loss from soil to water (e.g.Withers,P.J.A.,Dils,R.M.,Hodgkinson,R.A.,1999.Transfer of phosphorus from small agricultural basins with variable soil types and land use.p.41.In:Impact of Land use change on nutrient loads from different sources.IAHS publication no.257;Daly,K.,Mills,P.,Coulter,B.,McGarrigle,M.,2002.Modelling phosphorus concentrations in Irish rivers using land use,soil type,and soil phosphorus data.J.Environ.Qual.,31:590–599).However,previous studies quantifying the effect of STP and soil type on P solubility have usually relied on laboratory analyses of dried soil samples to quantify P solubility.Drying soil samples has been found to substantially increase P solubility (e.g.Bartlett,R.,James,B.,1980.Studying dried,stored soil samples —some pitfalls.Soil Sci.Soc.Am.J.,44:721–724;Turner,B.L.,Haygarth,P.M.,2001.Phosphorus solubilization in rewetted soils.Nature,411:258–258),and microbial biomass and organic matter (OM)have been implicated in this (e.g.Chepkwony,C.K.,Haynes,R.J.,Swift,R.S.,Harrison,R.,2001.Mineralization of soil organic P induced by drying and rewetting as a source of plant-available P in limed and unlimed samples of an acid soil.Plant Soil,234:83–90;Turner,B.L.,Driessen,J.P.,Haygarth,P.M.,McKelvie,I.D.,2003.Potential contribution of lysed bacterial cells to phosphorus solubilisation in two rewetted Australian pasture soils.Soil Biol.Biochem.,35:187–189).Many grassland soils in western Ireland contain high OM and moisture contents,and may therefore be particularly susceptible to drying-induced soluble P release.This study quantifies drying-induced changes in P solubility,by measuring water-extractable P (P w )and desorbable P (P feo )contents in moist and dried samples of a range of 33,mainly grassland,soils from western Ireland.Molybdate analyses of P w extracts before and after acid digestion were used to quantify dissolved reactive P (P w DRP),taken to represent predominantly readily soluble inorganic P,and dissolved molybdate-unreactive P (MUP),taken to represent predominantly readily soluble organic P.Dried soil samples were analysed for a wide range of general and P-specific characteristics,including STP (Morgan P and Olsen P)and the degree of sorption saturation (DPSS).Based onOMGeoderma 136(2006)120–135Abbreviations:Alox,ammonium-oxalate extractable aluminium;DDW,double distilled water;DPSS1,degree of phosphorus sorption saturation calculated using P ox and PSC1;DPSS2,degree of phosphorus sorption saturation calculated stoichiometrically using P ox and PSC2;Fe ox ,ammonium-oxalate extractable iron;MC,moisture content;OM,organic matter;P feo ,iron-oxide strip desorbable phosphorus;P i ,inorganic P;P o ,organic P;P ox ,ammonium-oxalate-extractable phosphorus;PSC1,phosphorus sorption capacity calculated as sum of PSI and P ox ;PSC2,phosphorus sorption capacity calculated stoichiometrically from Al ox and Fe ox ;PSI,phosphorus sorption index (remaining phosphorus sorption capacity);P w DRP,water-extractable dissolved reactive phosphorus;P w MUP,water extractable (dissolved)molybdate-unreactive phosphorus;P w TDP,water-extractable total dissolved phosphorus;STP,soil test phosphorus;SOC,soil organic carbon;TEB,total exchangeable bases.⁎Corresponding author.Department of Botany,Trinity College,University of Dublin,Dublin 2,Ireland.Tel.:+35316083068.E-mail address:dstyles@tcd.ie (D.Styles).0016-7061/$-see front matter ©2006Elsevier B.V .All rights reserved.doi:10.1016/j.geoderma.2006.03.017content,soils were divided into peat(>30%OM)and predominantly mineral(<30%OM)soils after Daly et al.(2000).Almost all soils exhibited large increases in P solubility after drying,with the size of drying-induced soluble P increases increasing in the order P w DRP<P w MUP<P feo.Absolute drying-induced increases were statistically similar between peat and mineral soil groups(e.g.P feo mean increases of9.06and13.81for peat and mineral soil groups,respectively).However,when expressed as a proportion of moist sample P solubility,all soluble P increases were significantly greater for peat compared with mineral soils(e.g.P feo percentage increases735%and155%for peat and mineral soil groups,respectively).Consequently,some differentiation in P solubility between peat and mineral soil groups was lost after drying.Drying-induced P w increases appeared to be related to soil OM and moisture contents,indicating drying-induced P release through microbial cell lysis and OM destabilisation.Increases were not related to moist sample P solubility or STP,resulting in the loss of some differentiation in P solubility between high and low STP soils.Drying-induced P feo increases were positively related to DPSS,reflecting chemical equilibrium control of desorbable P released from dried samples.It was concluded that the standard practice of drying soils prior to P solubility analyses reduces the inferred importance of soil type and STP as risk factors in diffuse soluble P losses.©2006Elsevier B.V.All rights reserved.Keywords:Soils;Soil drying;Phosphorus solubility;Soil test phosphorus;Peat;Ireland1.IntroductionDiffuse losses of phosphorus(P)from soil to water have been implicated in eutrophication of freshwaters, and developing reliable risk indicators for diffuse soil P loss has become urgent for EU member states who must comply with strict water quality guidelines arising from the EU Water Framework Directive(CEC,2000). One important risk factor for diffuse P loss is soil test P (STP)as measured by agronomic soil P tests(e.g. Mozaffari and Sims,1994;Foy et al.,1995;Smith et al.,1995;Withers et al.,1999;Gburek et al.,2000).In the Republic of Ireland,Morgan P(sodium acetate at pH4.8)is the standard STP method,while in a number of other European countries,Olsen P(0.5molar sodium hydrogen carbonate at pH8.5)is the standard STP method.Both water-extractable P(P w)and iron-oxide strip P(P feo)were originally developed,and are still used in some countries,as agronomic STP methods;other countries use them for environmental testing.These tests may be taken to represent the risk of soluble P loss from soils,and have been successfully related to soluble P losses in the field(Sharpley et al., 1981;Yli-Halla et al.,1995;Pote et al.,1999b; McDowell and Sharpley,2001;Torrent and Delgado, 2001).P w was initially developed as a simple and soil-type universal agronomic P test by Van der Paauw(1971). Soluble P extracted in this test is usually operationally defined using filtration through0.45µm pores,though considerable quantities of fine colloidal P<0.45µm remain in solution and may be measured during subsequent P analysis(Haygarth et al.,1997;Matthews et al.,1998).P w may then be further classified according to reaction with acidic molybdate reagent in molybdate reactive P(MRP)and defined as‘dissolved reactive P’(DRP)or‘molybdate-unreactive P’(MUP)accordingly. DRP is considered to be predominantly soluble inorganic P(P i),though it may include significant quantities of organic P(P o)and condensed P hydrolysed during acidic MRP analysis(Rigler,1968),in addition to fine colloidal P.Total dissolved P(TDP)is determined by MRP analysis on filtered samples in which organic and condensed P(MUP)have been digested,and includes fine colloidal P.In this paper,P w fractions are referred to as P w DRP,P w MUP and P w TDP. Unfortunately,as outlined above,these operational definitions do not correspond precisely with theoretical definitions of soil P fractions.For comparative purposes in this paper,it is assumed that P w DRP represents predominantly water-soluble P i,P w MUP represents predominantly water-soluble organic and condensed P, and P w TDP represents the sum of these fractions.The P feo test was developed by H.A.Sissingh,and published by Van der Zee et al.(1987).It is used as an indicator of desorbable P i,and involves shaking soil solutions with an iron-oxide-impregnated paper strip, which acts as a constant sink for P i.However,some P o species also adsorb onto the iron-oxide strips,such as inositol hexakisphosphate,and these may be hydrolysed during acid extraction of the strip and subsequent MRP analysis of the extracts.Robinson and Sharpley(1994) found that considerable quantities of P o were extracted by the P feo test.It is assumed here that P feo measures predominantly desorbable P i,though also includes some desorbable P o.For convenience,soils are usually dried in prepara-tion for laboratory analyses,despite evidence that drying and rewetting soils alters their physical and biological characteristics and releases soluble P(Bartlett and121D.Styles,C.Coxon/Geoderma136(2006)120–135James,1980;Pote et al.,1999a;Turner and Haygarth, 2001;Styles et al.,2006).Large decreases in the number of viable microbial cells present in soils immediately after drying and rewetting(Stevenson,1956;Sparling et al.,1985;Kieft et al.,1987;Van Gestel et al.,1991; Turner et al.,2003)have been attributed to cell lysis induced by osmotic shock upon rewetting with a low solute solution such as water(Kieft et al.,1987). Increases in P w after drying have been found to be dominated by the MUP fraction(Turner and Haygarth, 2001;Turner et al.,2003),and increases in this fraction have been found to correlate with soil microbial P content(Sparling et al.,1985;Turner and Haygarth, 2001;Turner et al.,2003).Microbial activity has also been implicated in the release and transformation of P associated with organic matter(OM),through mineral-ization flushes observed after dried soils were rewetted (Stevenson,1956;Bartlett and James,1980;Van Gestel et al.,1991).These mineralization flushes were attributed to high concentrations of dissolved soil organic C(SOC)in rewetted soils.Bartlett and James (1980)found that SOC became increasingly soluble after drying,with increasing storage time,and attributed this effect to oxidation of organic compounds.Thus, oxidation of SOC caused by soil drying and storage may directly release associated P o,and indirectly release P i via stimulated mineralization.The size and composition of drying-induced bicar-bonate-extractable P(similar to Olsen P)increases (Brookes et al.,1982;Sparling et al.,1985;Srivastava, 1997;Turner and Haygarth,2003)led Turner and Haygarth(2003)to conclude that mechanisms other than microbial P release were responsible—specifically, solubilisation of OM through physical disruption of OM coatings.Other physicochemical processes such as oxidation and hydrolysis have been implicated in drying-induced release of SOC and elements associated with P-binding(Al,Ca,Fe and Mn)in soils(Raveh and Avnimelech,1978;Bartlett and James,1980;Ross et al., 1994;Phillips and Greenway,1998).Drying-induced increases in the inositol hexakisphosphate concentra-tions of water extracts from five Australian pasture soils also suggest physicochemical mechanisms of P release (Turner and Haygarth,2003).This organic P fraction behaves similarly to P i in forming strong complexes with Al,Fe and Ca compounds.Drying soils may also result in dissolution and desorption from specific surface areas exposed by aggregate and OM breakdown (Raveh and Avnimelech,1978;Olsen and Court,1982; Chepkwony et al.,2001).Some studies have found that acidic STP extraction methods are unaffected by drying soils(Pote et al.,1999a;Olde Venterink et al.,2002).Large drying effects on P solubility analyses could obscure relation-ships with STP and soil type,as used to develop soil soluble P loss indices.Although samples maintained at sampled moisture content may undergo some biochemical changes,and physical changes after moist sieving(e.g.Chapman et al.,1997b),they are likely to better represent soils in original field conditions compared with artificially dried samples. Irish grassland soils,with a high proportion of peats and gleys,typically maintain high moisture contents (MC)throughout much of the year and may be particularly susceptible to laboratory drying effects. Further understanding the effects of soil drying on P solubility is important in order to reliably relate abundant STP data,and soil management plans,to diffuse P loss risk.While the primary aim of work conducted in this study was to develop soil P desorption coefficients for use in the Mask catchment of western Ireland(Styles et al.,2006),a secondary aim evolved to examine the importance of soil drying.Therefore,although no experiments were designed to identify specific causes of drying effects on soil P solubility,the substantial quantity of data collected in this study enabled some empirical observations to be made.Thus,the aims of this paper are:(1)To quantify the effects of sample drying on Psolubility,as measured by P w and P feo,for highOM and MC Irish grassland soils.(2)To relate drying induced changes in differentsoluble P fractions with soil type and soilcharacteristics,in order to infer causal mechan-isms of drying-induced P release and assess thepredictive power of soil characteristics for drying-induced soluble P release.(3)To assess the implications of drying soil samplesfor the interpretation of environmental laboratoryP tests,and relationships with STP.2.Materials and methods2.1.Soil samplingThirty grassland fields and three forested areas were selected within the Mask catchment of County Mayo, western Ireland,to include a range of soil types and soil P levels(Table1).At each sampling location,a composite soil sample was systematically collected in a‘W’pattern across the area,composed of approxi-mately25cores of2cm diameter and5cm depth.122 D.Styles,C.Coxon/Geoderma136(2006)120–135Samples were stored at 4°C prior to being sieved through a 4mm brass sieve within 3days of collection.Sieved,homogenised soils were then divided into two sub-samples,‘moist ’and ‘dried ’.Moist samples were stored in sealed foil trays at 4°C in order to maintain them at sampled moisture content and minimise biochemical reactions prior to analyses over the succeeding few days,while the remaining sub-samples were spread within folded paper to dry at approxi-mately 25°C for at least 10days.Dried soils were then 2mm mesh sieved and stored in foil trays prior to analyses.Immediately upon sieving,sub-samples of the moist soils were analysed for MC by weight loss after 24h at 105°C.Moist soils were weighed out on an equivalent oven-dry weight basis for analysis,while air-dried soils were weighed out and small adjustments made in final calculations to correct for remaining moisture content.2.2.Expression of resultsAll values are the mean of duplicate analyses,except pH,total organic carbon (TOC)and total nitrogen (TN)(no replicates).All results were corrected for moisture content and expressed on an oven-dry weight basis,prior to conversion to a volume basis using the empirical relationship between OM and bulk density reported by Jeffrey (1970):BD ¼1:482−0:6786log X ðr ¼−0:905Þ;where BD is expressed as g ml −1and X represents percentage ignition loss at 500°C (OM content).While it is more usual for soil concentrations to be expressed on a weight,rather than volume,basis,volume-based expression is more appropriate for ecological compar-isons (Jeffrey,1970),especially among soils of differing bulk densities.It also more accurately reflects soil –Table 1Information on soil group (P=peat,M=mineral),major characteristics (organic matter,moisture content,total exchangeable bases,pH),soil test P (Morgan P and Olsen P)and P saturation (DPSS1and DPSS2)for the studied soils Soil no.Group OM (%)MC (%)TEB (meq)pH Pm (mg l −1soil)Olsen P (mg l −1soil)DPSS1(%)DPSS2(%)1P 63.269.98.8 5.1 2.9 4.114.0 3.42M 13.441.4 4.6 5.27.553.843.711.23M 18.650.012.2 6.419.043.363.221.94M 18.255.521.07.5 6.511.663.814.15M 13.045.812.9 6.57.015.046.37.16P 50.576.2 3.3 4.8 2.5 3.97.6 1.07M 20.444.817.8 5.9 2.313.822.5 2.68M 11.440.919.77.413.926.551.110.69P 69.473.610.0 5.6 4.6 2.418.3 3.710M 18.148.79.8 5.710.137.658.614.611M 11.748.316.57.6 1.526.039.38.712M 10.241.9 5.3 5.4 2.621.532.1 5.514M 16.550.911.1 5.6 3.613.734.5 6.315M 17.345.015.0 6.7 2.7 6.228.4 4.616M 16.747.312.8 6.0 4.210.734.9 6.517P 38.961.425.77.4 2.7 2.618.6 2.918M 24.051.114.3 6.158.961.787.636.019M 17.249.422.37.423.634.556.113.120M 17.444.019.0 6.124.853.379.719.721M 17.242.018.1 6.529.265.176.422.923M 15.138.419.6 6.4 1.0 4.613.6 1.624P 47.162.217.2 5.5 2.7 3.924.1 4.525M 15.945.613.3 5.7 2.514.929.2 5.126P 38.072.012.4 6.0 2.910.930.1 5.827M 23.059.2 6.8 5.2 5.532.243.48.028M 16.146.98.8 5.514.063.466.622.129M 12.344.8 6.8 5.10.5 5.5 5.40.830M 10.437.9 6.0 5.2 2.922.133.1 5.031M 15.352.6 4.7 5.29.642.641.210.333P 54.374.49.9 5.3 4.18.117.1 3.134M 23.256.223.87.1 1.1 5.923.4 3.036M 27.059.016.4 6.3 6.717.942.08.637M18.952.317.86.833.050.475.422.3Concentrations expressed on an oven dry,volume basis (except MC,expressed on a wet-weight basis).123D.Styles,C.Coxon /Geoderma 136(2006)120–135water interaction,which occurs predominantly within a few centimeters of the surface(Sharpley,1985)and adjacent to preferential flow routes.Daly et al.(2000) found that soil OM content corresponded well with major Irish soil types,and in this study their definition of peat soils(OM>30%)is used.This boundary effective-ly divided the soils into predominantly mineral soils (≤27%OM)and peat soils(≥38%OM)(Table1). However,the mineral soil group does contain soils with relatively high OM contents and that,while predomi-nantly composed of mineral material,may be defined as peaty complexes.2.3.General soil analysesSoil pH was determined by stirring10g of dried soil with25ml double distilled water(DDW)and standing for30min prior to pH measurement with a calomel-glass electrode.Carbonate content was determined titrimetrically using NaOH following equilibration with HCl(Allen,1989).TOC and TN were analysed by dry combustion on a LECO CNS-1000elemental analyser after pre-treatment with sulphurous acid to burn off any carbonates.OM was determined by loss on ignition,after combustion at550°C for4h. Exchangeable bases and Mn were determined by extracting soils with ammonium acetate at pH7.0 (Allen,1989),while ammonium oxalate-extractable aluminium(Al ox),iron(Fe ox)and phosphorus(P ox) were quantified following Uusitalo and Tuhkanen (2000).Extraction solutions were filtered through Whatman No.1filter papers prior to measurement of elemental concentrations using a Perkin-Elmer®atom-ic absorption spectrophotometer.2.4.Soil P testsOlsen P was measured according to the method of Olsen et al.(1954).Soils were extracted at a soil/ solution ratio of1:20with0.5M NaHCO3at pH8.5, filtered through Whatman No.1filter papers,and the extracts acidified to pH5with H2SO4.Morgan P was determined by extracting6ml soil with30ml sodium acetate at pH4.8for30min,and measuring MRP in Whatman No.2filtrates(Morgan,1941).Remaining P sorption capacity of the soils(P sorption index:PSI)was determined according to the method of Freese et al. (1992).MRP remaining in solution was measured after shaking0.5g dried soil for24h with50ml0.02M KCl solution containing15mg P l−1as KH2PO4.Phosphorus sorption capacity(PSC)was calculated in two ways.Firstly,P ox was added to the PSI for each soil to give PSC1(mg l−1soil).Secondly,the sum of molar Al ox and Fe ox concentrations were multiplied by an activity factor of0.5to give PSC2(mmol l−1)(Freese et al.,1992).Degree of phosphorus sorption saturation (DPSS)was calculated using the PSC values.P ox, expressed on a volume and molar basis,was divided by volume and molar PSC1and PSC2values,respectively, to give DPSS1and DPSS2(Freese et al.,1992).2.5.P solubility analysesMoist and dried samples of all soils were analysed for P w by shaking1g of dry-weight equivalent soil with 100ml of DDW for30min,filtering through0.45µm filter papers,and analysing solution for MRP before and after acid-persulphate digestion(Grasshoff et al.,1999) to give soil contents of P w DRP and P w TDP,respectively. P w MUP was determined as the difference between these two values.P feo was determined according to the method of Menon et al.(1989)by shaking1g of dry-weight equivalent soil with40ml of solution(0.02M KCl was used instead of0.01M CaCl2),containing an iron-oxide impregnated Whatman No.54filter-paper strip2cm wide by10cm long,for16h.The paper strip was then removed,rinsed,and shaken in0.1M H2SO4for2h,and the MRP in this solution was analysed.All solution MRP concentrations were measured using the modified ascorbic acid method of John(1970).On moist samples, solubility analyses were carried out within2days of sieving in order to minimise storage changes.2.6.Statistical analysesData were statistically analysed using Data Desk version 6.0,checked for normal distribution,and parametric or non-parametric statistics used accordingly. Pearson-Product Moment correlations and least-squares regressions were used to test for the strength of associations and suspected causal effects,respectively, while paired t-tests were used to compare treatments (moist or dried samples)and different soluble P fractions.Mann–Whitney U-tests were used to compare soil groups(peat or mineral soils).Stepwise multiple regression analyses were performed after Velleman (1993),to derive predictive models for drying effects.3.Results3.1.Water-extractable PThe quantities of P w DRP and P w TDP extracted from moist and air-dried soil samples,and the drying-induced124 D.Styles,C.Coxon/Geoderma136(2006)120–135changes,expressed on a volume basis and as a percentage (for change),are displayed in Table 2.Moist sample P w TDP ranged from 1.15to 33.74mg l −1soil,and air-drying increased the quantities of P w TDP extracted by between 2.01mg l −1(soil 17)and 23.64mg l −1(soil 3),with a mean change for all soils of 9.37mg l −1.Only soil 7displayed a decrease in P w TDP after drying,driven by a decrease in P w DRP content,and this same soil displayed the smallest drying-induced P feo increase of any soil.Fig.1displays mean moist and dried sample soluble P fractions.Drying samples resulted in signif-icantly (paired t -test p <0.0001)greater release of P w MUP than P w DRP (all-soil mean increases of 6.33and 3.04mg l −1for P w MUP and P w DRP,respectively).Expressed as a proportion of moist sample contents,the mean P w MUP increase was over three times greater than the mean DRP increase (469%compared with 144%).Consequently,the mean proportion of P w TDP accounted for by P w MUP rose from approximately one quarter in moist samples to almost half in dried samples (Fig.1).Expressed on a soil-weight basis for the purpose of comparison with other studies,mineral soil P w MUP increases ranged from 0.96to 27.07mg P kg −1,and averaged 10.13mg P kg −1.Moist and dried sample P w DRP and TDP contents were strongly linearly correlated (e.g.moist and dried sample P w DRP r =0.96,p <0.0001),but moist and dried sample P w MUP contents were not significantly correlated (Table 3).This is displayed graphically in Fig.2a,which also highlights the linearity of the relationship between moist and dried sample P w DRP.Relative increases for each soluble P fraction afterTable 2Mean and S.D.of 2replicates for moist sample P w DRP,and P feo ,with change after drying (expressed as percentage change in brackets)Soil no.P w DRP P w TDP P feo MoistChangeMoist ChangeMoistChange(%)(mg l −1soil)(%)(%)10.98±0.10 3.10(316) 1.93±0.079.26(480) 4.05±0.0513.76(340)27.51±0.15 4.01(53)10.34±0.0017.30(167)21.53±2.0413.65(63)311.09±1.589.20(83)13.65±1.8223.64(173)31.89±3.2125.97(81)4 2.27±0.37 3.41(150) 4.04±0.5420.38(505)9.13±0.4221.96(241)5 6.74±0.66 2.23(33)9.36±0.8712.81(137)15.34±1.0715.35(100)60.69±0.01 4.74(689) 1.15±0.1011.61(1010)0.29±0.089.85(3378)710.06±0.54−5.81(−58)14.51±0.37−5.24(−36)20.46±2.00 1.28(6)8 6.57±0.86 1.69(26)10.09±0.90 6.39(63)18.22±0.9711.35(62)9 2.01±0.30 3.77(188) 2.77±0.268.46(306) 6.66±1.117.60(114)1010.23±0.97 6.00(59)12.83±1.0112.01(94)32.48±0.8126.10(80)110.91±0.19 1.92(211) 2.13±0.087.15(336) 3.58±0.568.96(250)12 2.89±0.19 2.95(102) 5.30±0.32 6.97(131)8.95±0.1910.33(116)14 1.39±0.00 6.41(462) 3.30±0.3511.55(350) 5.66±1.3624.28(429)15 3.37±0.07 1.15(34) 5.31±0.329.11(172)13.30±0.75 2.74(21)16 2.79±0.81 4.04(145) 5.45±1.7910.60(195)17.12±0.9910.57(62)170.43±0.01 1.38(322) 1.47±0.07 2.01(137) 1.58±0.01 6.21(394)1831.19±0.87 2.39(8)33.74±0.808.55(25)72.51±0.39−32.85(−45)197.29±0.10 1.35(18)9.52±0.327.59(80)21.03±0.7112.39(59)2015.19±1.63 2.73(18)18.24±1.65 6.69(37)50.22±1.6416.62(33)2114.77±0.56 2.95(20)17.13±0.468.63(50)47.46±1.8215.91(34)230.89±0.19 1.34(151) 2.85±0.25 4.87(171) 1.44±0.00 6.21(433)24 1.29±0.26 4.12(319) 2.37±0.417.30(308) 3.10±0.6013.03(420)25 3.18±0.12 2.19(69) 5.72±0.097.15(125)10.93±0.278.78(80)26 1.44±0.48 1.22(85) 2.60±0.47 4.52(174) 3.78±0.24 4.21(111)278.30±0.64 5.54(67)11.10±0.4113.57(122)16.85±0.1322.96(136)2813.27±1.58 2.49(19)16.41±1.90 6.87(42)26.37±2.8023.26(88)29 1.01±0.180.81(81) 2.13±0.19 3.33(156) 1.19±0.19 2.52(211)30 4.28±0.38 1.64(38) 6.70±0.18 4.40(66)11.29±0.48 6.76(60)317.90±0.107.73(98)10.23±0.5812.03(118)16.49±0.4721.19(129)330.80±0.22 4.62(579) 1.32±0.049.08(688) 2.24±0.908.74(390)340.72±0.20 2.23(311) 4.44±0.857.86(177)0.79±0.017.46(948)36 5.38±0.64 1.51(28) 5.78±1.278.25(143)12.16±0.3511.76(97)3712.32±0.075.19(42)14.84±0.0914.29(96)36.93±1.0816.92(46)Concentrations expressed on an oven dry,volume basis.125D.Styles,C.Coxon /Geoderma 136(2006)120–135drying were significantly negatively correlated with moist sample contents,while absolute increases were significantly positively correlated with dried sample contents,most strongly for P w MUP (r =0.98,p <0.0001).Fig.3displays the non-linear inverse relationships between moist sample soluble P contents and drying-induced percentage increases,best described by power equations.The P w MUP fraction was unique in displaying a positive correlation between dried sample content and drying-induced percentage increase (r =0.47,p ≤0.0001),and between absolute and relative increases (r =0.60,p <0.01)(Table 3).3.2.Desorbable PA greater quantity of P was desorbed to the iron-oxide strip over 16h than was released to water over 30min.Moist sample mean P feo and P w TDP were 16.52and 9.37mg l −1,respectively.Moist sample P feo ranged from 0.79mg l −1for soil 34to 72.51mg l −1for soil 18(Table 2).When respective decreases (P w TDP,soil 7,and P feo ,soil 18)were omitted,the all-soil mean drying-induced P feo increase of 12.77mg l −1was significantly greater than the all-soil mean P w TDP increase of 9.82mg l −1(paired t -test p =0.002).From here on,these two decreases are omitted from statistical descrip-tions and figures.Mean drying-induced relative increases were proportionately similar (272%compared with 206%;paired t -test p =0.45).As with the P w fractions,relative drying-induced increases in P feo were inversely correlated with moist sample desorption (Fig.3c),while actual increases were positively correlated with dried sample desorption (Table 3).P feo contents inmoist and dried samples were strongly linearly related (Fig.2b).3.3.Relationships with soil characteristicsThere were no particularly strong correlations between soluble P increases and soil characteristics (Table 3).Table 3shows that the most consistent correlations between soluble P increases and general soil characteristics involved MC and OM content (and related variables,such as TOC and TN),although MC and OM were strongly co-correlated (r =0.90,p ≤0.0001).Relative increases were positively correlated with MC and OM content for all soluble P fractions except P w MUP (close to statistical significance).Moist sample P w MUP,P w TDP and P feo were negatively correlated with MC.V olume-expressed increases were not significantly correlated with either MC or OM content when all soils were considered,but when peat soils were omitted from statistical analyses,most volume-expressed increases were weakly but significantly correlated with MC and OM (Table 4).Other significant correlations of note in Table 3are relatively strong positive correlations for DPSS (1and 2)with P feo increases,and negative correlations for DPSS (1and 2)with relative increases in P w DRP and (for DPSS1only)P w TDP and P feo .These negative correlations were stronger for the empirical DPSS1(based on P ox and PSI),and may reflect positive associations between DPSS and moist sample soluble P content.P feo increases were significantly positively correlated with STP (Olsen P,Morgan P and P ox )and extractable K,and significantly negatively correlated with PSI,while P w DRP increases weresignificantlyFig.1.Water-extractable DRP and MUP fractions before and after drying,averaged for all soils,for mineral soils and for peat soils.Error bars indicate S.D.126 D.Styles,C.Coxon /Geoderma 136(2006)120–135。