Study of Multilayer Flow Insulation Utilizing Open-Cellular Porous Plates
图像分割的误差分散半调算法
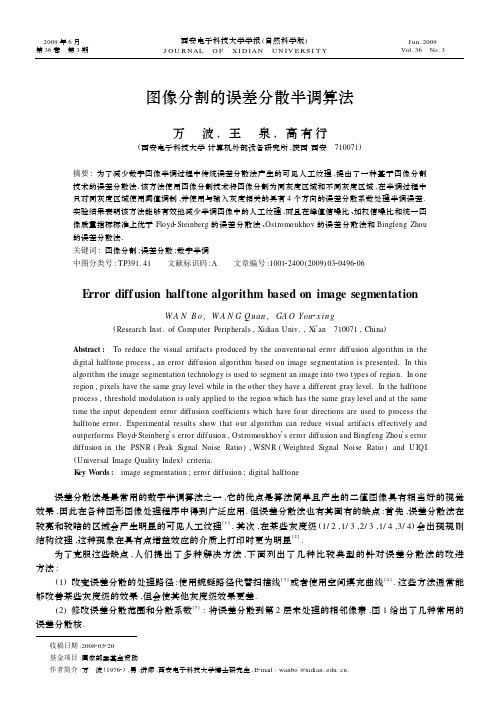
收稿日期:2008203220基金项目:国家部委基金资助作者简介:万 波(19762),男,讲师,西安电子科技大学博士研究生,E 2mail :wanbo @.图像分割的误差分散半调算法万 波,王 泉,高有行(西安电子科技大学计算机外部设备研究所,陕西西安 710071)摘要:为了减少数字图像半调过程中传统误差分散法产生的可见人工纹理,提出了一种基于图像分割技术的误差分散法.该方法使用图像分割技术将图像分割为同灰度区域和不同灰度区域,在半调过程中只对同灰度区域使用阈值调制,并使用与输入灰度相关的具有4个方向的误差分散系数处理半调误差.实验结果表明该方法能够有效地减少半调图像中的人工纹理,而且在峰值信噪比、加权信噪比和统一图像质量指标标准上优于Floyd 2Steinberg 的误差分散法、Ostromoukhov 的误差分散法和Bingfeng Zhou 的误差分散法.关键词:图像分割;误差分散;数字半调中图分类号:TP391.41 文献标识码:A 文章编号:100122400(2009)0320496206Error diff usion halftone algorithm based on image segmentationW A N B o ,W A N G Quan ,GA O You 2x i n g(Research Inst.of Computer Peripherals ,Xidian Univ.,Xi πan 710071,China )Abstract : To reduce the visual artifacts produced by the conventional error diffusion algorithm in the digital halftone process ,an error diff usion algorithm based on image segmentation is presented.In this algorithm the image segmentation technology is used to segment an image into two types of region.In one region ,pixels have the same gray level while in the other they have a different gray level.In the halftone process ,threshold modulation is only applied to the region which has the same gray level and at the same time the input dependent error diff usion coefficients which have four directions are used to process the halftone error.Experimental results show that our algorithm can reduce visual artifacts effectively and outperforms Floyd 2Steinberg πs error diffusion ,Ostromoukhov πs error diff usion and Bingfeng Zhou πs error diff usion in the PSNR (Peak Signal Noise Ratio ),WSNR (Weighted Signal Noise Ratio )and U IQ I (Universal Image Quality Index )criteria.K ey Words : image segmentation ;error diff usion ;digital halftone误差分散法是最常用的数字半调算法之一,它的优点是算法简单且产生的二值图像具有相当好的视觉效果,因此在各种图形图像处理程序中得到广泛应用.但误差分散法也有其固有的缺点:首先,误差分散法在较亮和较暗的区域会产生明显的可见人工纹理[1].其次,在某些灰度级(1/2,1/3,2/3,1/4,3/4)会出现规则结构纹理,这种现象在具有点增益效应的介质上打印时更为明显[2].为了克服这些缺点,人们提出了多种解决方法,下面列出了几种比较典型的针对误差分散法的改进方法:(1)改变误差分散的处理路径:使用蜿蜒路径代替扫描线[3]或者使用空间填充曲线[4].这些方法通常能够改善某些灰度级的效果,但会使其他灰度级效果更差.(2)修改误差分散范围和分散系数[5]:将误差分散到第2层未处理的相邻像素,图1给出了几种常用的误差分散核.2009年6月第36卷 第3期西安电子科技大学学报(自然科学版)J OU R NAL O F XI D IAN U N IV E R S I T YJ un.2009Vol.36 No.3图1 常用误差分散核(其中x 表示待处理像素点)(3)变化的阈值:使用输入相关的阈值代替固定的阈值.Eschbach 和Knox[6]提出反比例相关性,使得固有的边界增强得到控制,但对结构纹理效应没有什么效果.图2 不同误差分散法的分散系数Ostromoukhov [7]在Floyd 2Steinberg 误差分散法的基础上提出了一种简单有效的变系数误差分散法.该算法的核心思想是对不同的灰度级使用不同的误差分散系数,并将Floyd 2Steinberg 算法的误差分散系数从4个减少到3个,从而提高了算法的效率.该算法的误差分散方向如图2所示.Zhou [8]等在Ost romoukhov 算法的基础上引入了阈值调制,在阈值调制的基础上进一步优化中灰度区的误差分散系数,使中灰度半调图像的傅里叶频谱更接近理想的“蓝噪声”频谱.文献[8]的算法在中灰度区域取得了较好的半调效果,但存在两个问题:(1)对图像中每个像素都引入白噪声随机扰动进行阈值调制,这种方法在灰度相同区域能够避免半调图像的结构纹理,但在灰度不同的区域由于白噪声的引入会降低输出图像的信噪比,并使输出图像变得杂乱.(2)文献[728]的算法都只使用了3个误差分散系数,虽然提高了算法速度,但由于误差分散所涉及的相邻像素较少,不能完全利用误差分散法的优点.1 基于图像分割的误差分散法笔者在文献[728]的基础上提出了一种基于图像分割的误差分散法.该算法做了两点改进:(1)首先对输入图像进行图像分割,将同灰度区域标识出来,在半调过程中只对同灰度区域进行阈值调制,而不同灰度区域则不进行阈值调制,以减少引入白噪声带来的图像质量损失.(2)误差分散过程中采用Floyd 的4个误差分散方向,如图2(a )所示,并利用文献[8]中的方法重新搜索误差分散系数集合.下面首先讨论如何使用基于区域生长的图像分割方法对输入图像进行区域划分以获得具有相同灰度的区域,然后给出搜索具有4个分散方向的最优化误差分散系数集合的方法,最后通过实验对笔者提出的算法、Floyd 算法、文献[7]以及文献[8]的算法进行比较.111 利用图像分割技术对图像进行区域划分图像分割的目标是将数字图像划分为若干个不相交的区域.区域生长是众多的图像分割算法中最常用的一种.其核心思想是从某个像素出发,按照一定的准则,逐步加入邻近像素,当满足一定条件时,区域生长终止.区域生长的算法描述如下:(1)对图像顺序扫描,找到第一个还没有归属的像素,该像素为(x 0,y 0).(2)以(x 0,y 0)为中心,考虑(x 0,y 0)的4个邻域像素(x ,y ),如果(x ,y )满足生长准则,则将(x ,y )与(x 0,y 0)合并,同时将(x ,y )压入堆栈.(3)从堆栈中取出一个像素,把它当作(x 0,y 0),回到步骤(2).(4)当堆栈为空时,当前区域生长结束,回到步骤(1).(5)重复步骤(1)~(4),直到图像中的每个点都属于某个区域时,生长结束.笔者使用区域生长法找出图像中需要使用阈值调制的区域,即灰度相同的区域.这些区域的生长准则是794第3期 万 波等:图像分割的误差分散半调算法相邻像素的灰度误差T =0.假设通过区域生长法将图像分割为N (N ≥1)个区域R 1,R 2,…,R N ,每个区域中像素个数为P 1,P 2,…,P N ,这些区域中P i 较小(例如,P i =1)的区域不能算作同灰度区域,因此需要根据P i 来进一步确认哪些区域需要进行阈值调制,当区域R i 的像素点个数P i 满足P i ≥M , i =1,2,…,N ,(1)时认为R i 是需要进行阈值调制的区域.其中M 为像素个数阈值,可以设定为不同的值,后面的实验中取M =8.在半调过程中只需要知道待处理像素是否在需要阈值调制的区域中,而不需要知道在哪个区域中,因此在图像分割完成后对图像进行扫描,根据式(1)进行判断得到一个区域分布矩阵M R ,即M R =011 00011...11011 (11)...............001...10001 (10) .在M R 中值为0的元素表示对应的像素不需要阈值调制,为1的元素表示对应的像素需要进行阈值调制,在半调时只需查找这个区域分布矩阵,对值为1的元素进行式(2)所示的阈值调制[8].t (g )=128+(rand (x ,y )mod 128)・m (g ) ,(2)其中t (g )是调制后的阈值,rand (x ,y )是白噪声随机数发生器,(x ,y )是待处理像素在输入图像中的位置,g ∈[0,255]是待处理像素在输入图像中的灰度级,m (g )∈[011,110]是阈值调制强度[8].112 搜索最优化误差分散系数集合当非周期结构的傅里叶频谱是放射对称且没有低频分量时,它被称为具有“蓝噪声”特性.半调理论认为,误差分散法的输出图像的傅里叶频谱越接近“蓝噪声”频谱,其质量就越好[9],因此搜索误差分散系数的目标是使输出图像的傅里叶频谱更接近“蓝噪声”.文献[728]都通过最优化的方法得到一组和输入灰度相关的3个方向误差分散系数,笔者利用他们的方法搜索一组4个方向误差分散系数.搜索过程用到的代价函数由两部分构成.第1部分引入了放射平均功率谱来衡量频谱的对称性:P 0(f r )=1N 0(f r )∑N 0(f r )i =1^P (f ) ,P 45(f r )=1N 45(f r )∑N 45(f r )i =1^P (f ) ,P 90(f r )=1N 90(f r )∑N 90(f r )i =1^P (f ) ,(3)图3 在频谱中沿0°,45°和90°3个方向的放射平均功率谱式中定义的3个放射平均功率谱分别是图3中0°,45°和90°3个方向的放射平均功率谱,然后将这3个方向的放射平均功率谱的相关性作为代价函数的第1部分.N 0(f r ),N 45(f r ),N 90(f r )分别表示在频谱上沿半径为r 的环面进行采样,落在垂直、对角和水平方向的频率采样数.P (f )是文献[9]中定义的频谱估计.第2部分衡量目标频谱和“理想蓝噪声频谱”的相似度.该相似度通过计算对称平均功率谱曲线P (f )主要频率覆盖的面积和整个曲线覆盖的面积之比得到,该比值称为低频比例,即L (g )=∫f gP (f )d f ∫1/21/2P (f )d f ,(4)其中g 表示灰度级.代价函数由这两部分的加权和构成:894 西安电子科技大学学报(自然科学版) 第36卷C (g )=C o (P (g )0,P (g )45,P (g )90)・w +L (g )・(1-w ) ,(5)其中g 是输入图像灰度,w 是权重(0≤w ≤1),C o 是3条曲线P (g )0,P (g )45,P (g )90的相关性函数,值越大相似度越小.有了式(5)的定义之后,搜索的目标就是对给定灰度g 找到使代价函数C (g )值最小的误差分散系数.表1是w =015时找到的关键灰度[7]的误差分散系数,0~127中其他灰度的误差分散系数可以通过平滑插值得到,而128~255灰度的误差分散系数可以通过对称方式求得,即灰度g 的误差分散系数和灰度255-g 的误差分散系数相同[7].表1中的误差分散系数即图2(a )中的N 10,N -11,N 01和N 11,实际半调过程中的误差分散系数e 10,e 11,e 01和e 11为e 10(g )=N 10(g )/M (g ) , e -11(g )=N -11(g )/M (g ) ,e 01(g )=N 01(g )/M (g ) , e 11(g )=N 11(g )/M (g ) ,M (g )=N 10(g )+N -11(g )+N 01(g )+N 11(g ) .(6)表1 关键灰度的误差分散系数关键灰度N 10N -11N 01N 11关键灰度N 10N -11N 01N 11067532106430512153312310916573512724223921243525112347757141414358334385453172134201454730795412017191042191723107422115202236122516023212762211926323421872861682 实验结果图4和图5给出了Floyd 误差分散、文献[8]的算法和笔者提出的算法对具有同一灰度(灰度级为64和128)的图像进行处理得到的半调图像以及半调图像的傅里叶频谱.从图4和图5可看出笔者提出的算法得到的半调图像纹理更少,而且半调图像的傅里叶频谱具有更好的放射对称特性,更接近理想的蓝噪声频谱.图6是用于测试的两幅图像.图7给出了文献[728]的算法和笔者提出的算法对图6中的两幅图像进行处理所得半调图像.从图7可以看出笔者提出的算法得到的半调图像纹理更少,图像更加均匀,效果更好.表2给出了图7的半调图像在峰值性噪比(PSNR )、加权性噪比(WSNR )、线性失真衡量(LDM )[10]和统一图像质量指标(U IQ I )[11]这些评价标准上的比较.从表2可以看出笔者提出的算法得到的半调图像具有比另两种算法更好的评价指标.表2 不同半调算法的评价标准比较图像算法PSNR WSNR LDM U IQ I Lena文献[7]的算法617203902619007000192490801071484文献[8]的算法617210002717027000192795801076980笔者提出的算法617505403011316000193849201085324Pepper 文献[7]的算法618981802613801000193523001121534文献[8]的算法619239802713096000193936901129727笔者提出的算法6196862029103640001944131011385003 总 结在文献[728]的基础上笔者对传统误差分散法进行了改进,首先利用区域生长法对输入图像进行图像分994第3期 万 波等:图像分割的误差分散半调算法图4 3种半调算法对灰度为64的图像进行处理的结果图5 3种半调算法对灰度为128的图像进行处理的结果割得到具有相同灰度的区域,然后在半调过程中只对灰度相同区域进行阈值半调,并使用了重新搜索的4个方向误差分散系数对半调误差进行处理.实验结果表明笔者提出的算法的性能优于文献[728]的算法以及传统的Floyd 误差分散法.05 西安电子科技大学学报(自然科学版) 第36卷图6 用于测试的两幅图像图7 不同半调算法产生的半调图像比较参考文献:[1]Knox K T.Evolution of Error Diff usion [J ].Journal of Electronic Imaging ,1999,8(4):4222429.[2]叶玉芬,郭宝龙,马佳.基于视觉差的误差扩散半色调算法[J ].计算机工程,2006,32(16):1952197.Ye Yufen ,Guo Baolong ,Ma Jia.Perceived Error 2based Error Diff usion [J ].Computer Engineering ,2006,32(16):1952197.[3]Ulichney R.Digital halftoning [M ].Cambridge :MIT Press ,1987.[4]Velho L ,G omes J.Digital Halftoning with S pace Filling Curves [C]//C omputer G raphics.New Y ork :ACM ,1991:81290.[5]Shiau J ,Fan Z.A Set of Easily Implementable Coefficients in Error Diffusion with Reduced Worm Artifacts [C ]//Proceedings of SPIE.Bellingham :Society of Photo 2Optical Instrumentation Engineers ,1996:2222225.(下转第546页)105第3期 万 波等:图像分割的误差分散半调算法645 西安电子科技大学学报(自然科学版) 第36卷4 结束语用同一旋转因子表实现了任意N=2n≤N max=2n max点的FF T处理,它的旋转因子地址产生与n的奇、偶性及当前处理长度无关,仅是最大可处理长度的函数,因此所需资源少,地址产生简单;文中算法使存储(见公式(2))的存储体在访问次数方面,比目前较好算法[4,627])有明显改善.W2n0N max参考文献:[1]Shin M,Lee H.A High2speed Four2parallel Radix224FFT/IFFT Processor for UWB Applications[C]//IEEEInternational Symposium on Circuits and Systems,ISCAS2008.Washington:IEEE,2008:9602963.[2]Lee H,Shin M.A High2Speed Low2Complexity Two2Parallel Radix224FFT/IFFT Processor for UWB Applications[C]//IEEE Asian Solid2State Circuits Conference,ASSCCπ07.K orea:IEEE,2007:2842287.[3]Han Wei,Erdogan A T,Arslan T.The Development of High Performance FFT IP Cores Through Hybrid Low PowerAlgorithmic Methodology[C]//Proceedings of Asia and South Pacific Design Automation Conference,ASP2DAC2005: Volume1.Shang Hai:IEEE,2005:5492552.[4]谢应科,付博.数据全并行FFT处理器的设计[J].计算机研究与发展,2004,41(6):102221029.Xie Y ingke,Fu Bo.Design and Implementation of High Throughput FF T Processor[J].Journal of Computer Research and Development,2004,41(6):102221029.[5]Jo B G,Sunwoo M H.New Continuous2flow Mixed2radix(CFMR)FFT Processor Using Novel In2place Strategy[J].IEEE Trans on Circuits and Systems,2005,52(5):9112919.[6]Li Xiaojin,Lai Z ongsheng.A Low Power and Small Area FFT Processor for OFDM Demodulator[J].IEEE Trans onConsumer Electronics,2007,53(2):2742277.[7]万红星,陈禾,韩月秋.并行数据FFT/IFF T处理器的设计[J].北京理工大学学报,2006,26(4):3382341.Wan Hongxing,Chen He,Han Qiuyue.Parallel Architecture FFT/IFFT Processor[J].Trans of Beijing Institute of Technology,2006,26(4):3382341.[8]唐江,刘桥.基于FP GA的基24FFT算法的硬件实现[J].重庆工学院学报(自然科学版),2007,21(3):82284.Tang Jiang,Liu Qiao.Hardware Implementation of Radix4FFT Algorithm Based on FP GA[J].Journal of Chongqing Institute of Technology(Natural Science Edition),2007,21(3):82284.[9]Jiang R M.An Area2Efficient FF T Architecture for OFDM Digital Video Broadcasting[J].IEEE Trans on ConsumerElectronics,2007,53(4):132221326.[10]Garrido M,Grajal J.Efficient Memoryless Cordic for FFT Computation[C]//IEEE International Conference onSpeech,Signal Processing and Acoustics,ICASSP2007:Volume2.Honolulu:IEEE,2007:1132116.(编辑:齐淑娟) (上接第501页)[6]Eschbach R,Knox K T.Error2diff usion Algorithm with Edge Enhancement[J].Journal of the Optical Society ofAmerica,1991,8(12):184421850.[7]Ostromoukhov V.A Simple and Efficient Error2Diff usion Algorithm[C]//Computer Graphics.New Y ork:ACM,2001:5672572.[8]Zhou Bingfeng,Fang Xifeng.Improving Mid2tone Quality of Variable2coefficient Error Diff usion Using ThresholdModulation[C]//ACM Trans on Graphics.New Y ork:ACM,2003:4372444.[9]Ulichney R A.Dithering with Blue Noise[J].Proc IEEE,1988(76):56279.[10]Valliappan M,Evans B L,Tompkins D A D,et al.Lossy Compression of Stochastic Halftones with JBIG2[J].ProcIEEE Int Conf on Image Processing,1999(1):2142218.[11]Wang Z,Bovik A C.A Universal Image Quality Index[J].IEEE Signal Processing Letters,2002,9(3):81284.(编辑:郭 华) 。
岩体非连续裂隙网络三维面状渗流模型
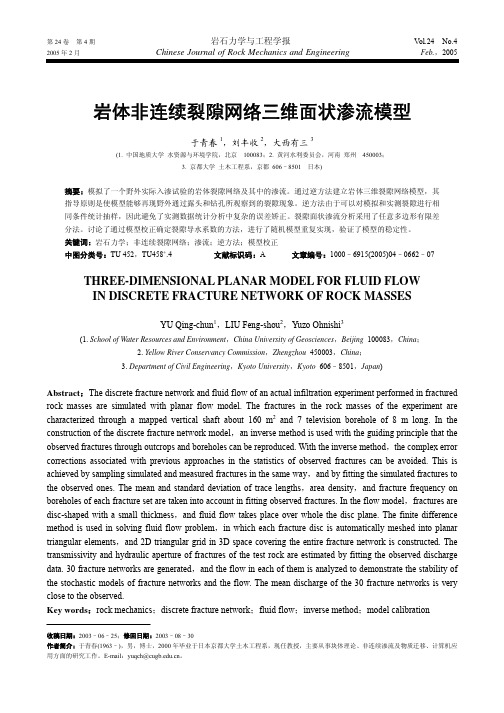
目前,模拟岩体非连续渗流的模型有 2 类:一 类是管状流模型(channel model);另一类是面状流 模型(planar model)。把单个裂隙看作粗糙板状几何 体是岩石力学的传统观点
[1,2]
。以野外观察到的裂
隙数据为基础,建立岩体三维不连续面网络模型, 在此基础上直接构筑三维空间中的面状数值网格进 行渗流分析是面状流模型的基本思路。除了边界和 力学参数的确定等所有三维模型所面临的共同困难 之外,算法复杂,计算量大是面状渗流模型的主要 困难。这也是目前管状流模型被较广泛采用,而面 状流模型用于野外实际渗流分析的实例还很少见的 主要原因[3
式中: q 为单位宽度上裂隙的流量, b 为裂隙张开 文[7]认为,对于粗糙裂隙,若在式(1)中加一个 修正系数 f 来反映裂隙表面的粗糙性,三次方定律 仍然适用,则式(1)变为 1 b3 q= ∆P f 12γ
cm),用以观测自地表通过岩体裂隙进入竖井的流 (2)
量。观测得到的稳定总流量为 3.29×10
~ 5]
2
野外试验数据
为了研究地下深处岩体的力学特征,日本核动
。作者认为,面状流模型在详细刻画
渗流通道方面具有管状流模型无法比拟的灵活性, 只要对不同的单元给定不同的渗透参数,面状流就 可以产生管状流效果。从这个意义上讲,管状流模 型可以看作是面状流模型的一种特殊情况。 在面状流模型中,单个裂隙的渗透性由三次方 定律计算[6],即
• 663 •
水试验得到的导水系数分摊到与此段钻孔相交的各
1
引
言
裂隙上去。这种方法的假设条件是非常苛刻的:裂 隙必须是无限大并与钻孔直交,裂隙之间不能交叉 或交叉之后对裂隙网络的导水能力无影响。 考虑到裂隙的有限性、相互交叉等影响因素, 要较准确的计算裂隙的导水系数,必须借助于非连 续裂隙网络模型。此模型无法得到简单的解析解, 必须通过数值法求解非连续裂隙网络渗流问题,并 通过模型的校正确定裂隙的渗流参数。本文结合一 个具体的野外实际渗流试验,探讨解决上述问题的 方法。
海上溢油运动数值模拟方法的探讨与改进

第3期2009年5月华东师范大学学报(自然科学版)Journal of East China Normal University (Natural Science )No.3 May 2009文章编号:100025641(2009)0320090208海上溢油运动数值模拟方法的探讨与改进3刘伟峰, 孙英兰(中国海洋大学环境科学与工程学院,山东青岛 266100)摘要:简单总结了海上溢油数值模拟的三种模型方法———油膜扩展模式、对流扩散模式和油粒子模式,并针对目前流行的油粒子模式在油膜自身扩展过程和扩散面积计算方面的不足,根据经典Fay 理论对其进行了补充和改进.提出的新模拟方法将溢油运动过程的分为自身扩展和紊动扩散两个阶段,前一阶段根据Fay 理论修正模式计算,后一阶段采用油粒子方法模拟,通过“油膜粒子化”技术将两阶段进行衔接.数值模拟实验结果表明,该方法因充分考虑了溢油初始阶段的自身扩展过程,能够弥补油粒子方法的不足,符合溢油在不同时期扩散机制亦不同这一实际情况.关键词:溢油; 数值模拟; 油粒子; 改进中图分类号:P 731.1 文献标识码:A 收稿日期:2008211 基金项目:青岛市海洋环境保护规划(HB 2005001) 第一作者:刘伟峰,男,博士研究生,研究方向为环境规划与管理.E 2mail :lwf _ouc @. 32008年河口海岸科学全国博士生学术论坛资助Study and improvement of oil spill simulation methodsL IU Wei 2feng , SUN Y in 2glan(of Environmental Science and Engineering ,Ocean University of China ,Qingdao S handong 266100,China )Abstract : Three oil spill models were briefly reviewed.And based on the analysis of problems and defects existing in “Oil Particle ”approach ,a “Two 2Step ”method was put forward for oil spill simulation.The method divides oil spill movement into two step s :self 2expansion and turbu 2lent expansion.The former step was handled by refinement of Fay ’s equations ,while the latter was handled by “Oil Particle ”approach.Numerical experiment results show the “Two 2Step ”method can make up the shortcomings of “Oil Particle ”approach and accords with the reality that spilled oil has different diff usion mechanism in the process of movement.K ey w ords : oil spill ; numerical simulation ; oil particle ; improvement0 引 言近几十年来,海上溢油频繁发生,严重影响了海洋的生态环境;韩国“12.7”特大海上溢油事故再次警示世人,溢油之祸不容忽视.溢油在海上运动变化的复杂性,使其成为当今海洋环境保护中最困难的问题之一.国内外相继开展了溢油方面的研究工作,用数学模型模拟第3期刘伟峰,等:海上溢油运动数值模拟方法的探讨与改进19和预测溢油时空分布的方法得到了广泛应用,海上溢油运动的数值模拟方法也在不断发展[1].本文在分析总结国内外溢油研究成果的基础上,对当今流行的模拟方法进行了改进,提出海上溢油运动数值模拟的新方法.1 国内外海上溢油运动数值模拟研究进展20世纪60年代,欧美等国家已开始对海上溢油进行预测,并发展了许多溢油模式[1].我国对海上溢油的研究始于20世纪80年代,许多学者曾对溢油摸式进行了研究,也取得较大进展[2].溢油在海面上的运动及变化是一个极其复杂的过程,受其物理、化学和生物等过程的影响,且与石油的性质、海洋水动力环境及海洋气象环境等密切相关.这些过程包括漂移、扩散、蒸发、溶解、乳化和沉降以及浮油和海岸线的相互作用等;其中漂移、扩散等动力学过程是国内外溢油研究的重点.归纳前人的研究成果,溢油运动的数值模拟方法可根据不同的数学模型计算理论分为油膜扩展模式、对流扩散模式和油粒子模式三类,以下对三类方法进行简要的分析与总结.1.1 油膜扩展模式该数值方法主要采用质心轨迹结合油膜扩展经验公式的方法,即通过扩展经验公式给出溢油面积的等效园(或椭圆),然后迭加到溢油质心漂移位置之上[3].对于油膜质心的漂移轨迹的确定,国内外各学者普遍都采用欧拉2拉格朗日追踪法,并考虑了风的影响,油膜质心的漂移速度为表面海流和风所引起的流速的矢量之和[4].在溢油扩展模型研究方面,Blokker只考虑重力和溢油体积的影响,得到了油膜扩展理论[5];Fay在这方面进行了开创性的工作,提出了油膜的三阶段扩展理论,认为油膜呈圆形扩展[6].Fay理论对于研究溢油扩展模型起到了很重要的推动作用,但因其基于静水假定,没有考虑风和流的影响,仅适用于静水或恒流条件下的油膜扩展[1].海上油膜扩展过程的一个明显特征是它的各向异性,因此后来的许多学者在Fay理论的基础上将油自身的扩展过程与海水扰动因素作用下的分散过程结合起来,建立了各种溢油扩展模型.如Mackay在Fay的第二阶段公式中加上风的影响并分别建立了厚油膜和薄油膜扩展的计算公式[7];刘肖孔等综合溢油三个阶段的扩展机理,提出了扩展尺度随时间统一变化的公式[8];W T Lehr,R T Fraga和M S Belen对Fay理论进行了修正,建立了油膜将在风向上被拉长的椭圆模型[9];赵文谦和武周虎同时考虑了油膜扩展和各向异性扩散作用,以及油膜边缘消失的过程,建立了扩散范围的数学模型[10].Fay理论的修正模型在一定程度上取得了较好的计算结果,早期得到了广泛的应用.该模式对溢油轨迹的计算比较准确,但油膜扩散面积的可靠性仍有不足.1.2 对流扩散模式该数值方法由二维有限流体动力学和油在溢出地的寿命分析所组成,将海上已知的溢油量或者根据环境影响评估得出一个浓度作为源项加入动力学方程,然后数值求解对流扩散方程[11,12].该方法的主要困难在于稳定性问题,即数值离散化过程中可能会引进与物理扩散无关的数值扩散,使得所得结果失真,不能描述真实的物理过程.另外,还有一些过程难以利用对流扩散方程来模拟,如比较典型的非Fick扩散问题.1.3 油粒子模式华东师范大学学报(自然科学版)2009年29油粒子方法最早是Johansen和Elliot等提出的[13,14],该方法通过把溢油分成许多离散的小油滴来模拟溢油在海水中的漂移扩散过程,可以直接模拟出扩散方程的实际物理现象,而不是去求解扩散方程.该方法正确解释了溢油在重力扩展停止以后的扩散现象物理学问题,突破了采用对流扩散方程模拟溢油的传统方法,可以更确切地表述溢油对各种海洋动力因素的响应过程,不仅避免了对流扩散模式本身带来的数值扩散问题,同时还可以正确重现海上油膜的破碎分离现象,能够准确地描述溢油的真实扩散过程.因此,它在溢油模拟发展过程中具有划时代的意义,也成为了当今流行的溢油模式.最近很多室内和现场实验都支持该方法,国内外很多学者对其进行了应用和发展,并得到了较理想的结果[15218].2 油粒子模式的缺陷分析2.1 紊动扩散模拟油膜自身扩展过程油粒子方法,是把浓度场模拟为由大量的粒子组成的“云团”,其中每一个粒子表征一定数量的示踪物质.模型粒子的平流过程具有拉格朗日性质,可用拉格朗日方法模拟.剪流和湍流引起的紊动扩散过程属于随机运动,可用随机走动法来实现模拟,亦即将湍流视为一种随机流场,而每个模型粒子在湍流场中的运动则类似于流体分子的布朗运动,由于每个粒子的随机运动而导致整个粒子云团在水体中的扩散过程.这种模拟方法实际上是确定性方法和随机性方法的结合,即采用确定性方法模拟平流过程,采用随机性方法模拟扩散过程.溢油发生后,油膜将在重力和惯性力的作用下迅速扩展开来,自身扩展是油膜的主要运动形式;随着油膜自身扩展的进行,油膜越来越薄,在风、浪、流的紊动作用下开始破碎、分散,紊动扩散成为油膜扩散的最主要的方式.油粒子模式能够准确地模拟出溢油在重力扩展停止以后的扩散现象,但也忽略了溢油初期重力和惯性力作用下的自身扩展过程.对于小规模溢油,油膜的自身扩展过程较短,初期扩展面积较小,对其作忽略处理而直接模拟溢油的紊动扩散过程对计算结果影响不大.但对于大规模瞬时溢油,如船舶折断,储运油品迅速泄出以及其它形式的溢油量较大,油品泄漏时间很短的情况,油膜面积将在短期内急剧扩大,随后扩延速度变慢;溢油初期的自身扩展效应显著大于湍扩散效应,因此对油膜初期的自身扩展过程作忽略处理,或者说用紊动扩散来模拟油膜的自身扩展过程就显的不够合理. 2.2 溢油量对扩散面积的影响不直观油粒子方法将油粒子定义为一些很小的圆球,直径分布在10~1000μm之间.考虑到油滴直径的变化范围,精确地表示一个溢油膜所需要的实际粒子数应是相当大的,要在计算机中同步地堆栈太多的粒子特性参数是不可能的.因此在实际计算中,一般根据计算机的容量和运行时间的长短来确定最大可能的粒子数目,用附加体积参数的方法来实现对油粒子特性的模拟,每个油粒子代表溢油体积的一部份.将某个油粒子的体积参数定义为V i,其所占油膜总体积的百分比为f i,则每个油粒子的特征体积为V i=f i・V0(其中,V0为溢油的初始体积)[13].这样,油粒子模式中不同规模的溢油量就通过油粒子总数和特征体积的不同来体现.实际情况下,海面上油膜的扩散面积将随溢油规模的不同而有较大差异.油粒子方法的计算结果是否如此,本文通过数值实验来进行分析.数值实验如下.环境条件 恒定流速u=0.5m/s,静风条件,暂不考虑油膜的挥发.情景条件 瞬时溢油,溢油量分别为10t,20t,100t,模拟时间为12h.计算参数 油粒子特征体积:V i=0.01t,对应的油粒子总数分别为1000,2000和第3期刘伟峰,等:海上溢油运动数值模拟方法的探讨与改进10000.紊动扩散系数取为定值:k α=k β=1.0m 2/s.数值实验结果如图1和图2所示.溢油的前几个小时内,10t ,20t ,100t 溢油量的瞬时扩散面积相差无几;整个模拟过程中,溢油量加倍,其扩散面积仅增大14%~18%;溢油量增大10倍,其扩散面积也仅增大24%~42%.图1 不同规模的溢油漂移扩散过程(油粒子法)Fig.1 Oil drifting and diff usion process of different amount (Oil Particle approach)图2 不同规模的溢油瞬时扩散面积随时间的变化(油粒子法)Fig.2 Computing results of instant diff usion area of spilled oil (Oil Particle approach )数值实验结果表明,油粒子方法计算的扩散面积受溢油规模的影响并不显著,与实际情况不相符.对溢油扩散面积计算的不足,就成了油粒子方法的另一个缺陷.3 油粒子模式的改进3.1 两阶段模拟法针对油粒子模式的不足,本文对其进行了补充和改进,提出了两阶段模拟的方法.该方法将溢油运动过程的分为自身扩展和紊动扩散两个阶段,前一阶段根据Fay 理论修正模式计算,后一阶段采用油粒子方法模拟,通过“油膜粒子化”将两阶段进行衔接.3.1.1 自身扩展阶段39华东师范大学学报(自然科学版)2009年(1)油膜扩展根据Fay 理论,油膜的自身扩展阶段即为溢油初期的重力———惯性力平衡阶段.该阶段内重力———惯性力占主导,由于油水密度差引起油膜加速塌落,形成油的初始运动,油膜扩展直径为[6]r (t )=c 1(ΔgV t 2)14,油膜自身扩展持续时间为t f =(c 2/c 1)4V 1/3(v ・Δg )-1/3.式中,V 为油膜体积,Δg 为约化重力加速度(Δg =(1-ρ0/ρw )g ,ρw 为海水密度,ρ0为油膜密度),v 为运动黏性系数,c 1,c 2均为经验常数,t 为时间.Fay 理论是建立在静水假定基础上的,认为油膜成圆形扩展;而实际海况下油膜扩展过程具有明显的各向异性特征[1],因此本文将Fay 模式的扩展直径加以订正,订正后的短轴仍按上述三式计算,长轴l 改为l =r +cw δt ε.其中,w 为风速;c ,δ,ε为经验常数,与油的种类、性质有关.(2)油膜漂移油膜质心的漂移轨迹采用欧拉2拉格朗日追踪法.在风和潮流的作用下,油膜中心初始位置S 0,经Δt 时间后漂移到了新的位置S ,其中S =S 0+∫t 0+Δt t 0V L d t ,式中V L 为拉格朗日速度.油膜中心的漂移速度和方向是表面海流和风所引起的流速之矢量和,即v 0=v w +αD ・v α.其中v 0为油膜中心漂移速度,v w 为海面流速,v α为海面10米处风速,α为风漂流系数,D 为引入漂流偏角的一转换矩阵[4].3.1.2 紊动扩散阶段(1)油膜粒子化为采用油粒子方法模拟,需将自身扩展阶段末的油膜转化为一系列的粒子.将油膜分割成N 个小单元,其中每个小单元代表溢油体积的一部份.根据油膜质心的位置(x 0,y 0),求出每个油粒子所在的位置(x i ,y i ,i =1,…,N ),如图3所示.根据计算机的容量和运行时间的长短来确定粒子总数N ,而用附加体积参数的方法来实现对油粒子特性的模拟.图3 油膜粒子化过程Fig.3 Process of changing oil slick into particles49第3期刘伟峰,等:海上溢油运动数值模拟方法的探讨与改进(2)油粒子方法根据确定的油粒子总数,采用当今流行的油粒子方法对紊动扩散阶段的溢油的运动进行模拟.3.2 计算结果分析采用两阶段法再次进行上面的数值实验.图4为模拟的100t 溢油的漂移扩散过程.由图中可以看出,油膜自身扩展持续时间约18min ;油膜自身扩展效应显著大于紊动扩散效应,因此油膜的扩散面积也显著大于油粒子方法的模拟结果.两阶段法充分考虑了油膜的自身扩展过程,弥补了油粒子方法的不足.图4 两阶段法与油粒子法计算结果的对比(100t )Fig.4 Results comparison between “Two 2Step “and “Oil Particle ”methods (100t )图5为两阶段法计算的不同规模的溢油漂移扩散过程,整个模拟过程中,溢油量加倍,其扩散面积增大0.23~0.85倍;溢油量增大10倍,其扩散面积增大2.0~3.4倍,如图6所示.两阶段法计算的扩散面积受溢油规模的影响较为显著,改进了油粒子方法对扩散面积计算不足的缺陷.图5 不同规模的溢油扩散范围随时间的变化(两阶段法)Fig.5 Oil drifting and diff usion process of different amounts (Two 2step method )59华东师范大学学报(自然科学版)2009年图6 不同规模的溢油瞬时扩散面积随时间的变化(两阶段法)Fig.6 Computing results of instant diff usion areas of spilled oil(Two2Step method)由于难以获得真实、完整的海上溢油资料,本文的计算结果未能用实测资料进行验证.海上溢油资料的相对缺乏,也是制约当前溢油研究发展的主要因素.鉴于油粒子方法的方法理论及计算结果已得到国内外专家的普遍认可,本文以油粒子方法为参照,用数值实验资料对两阶段模拟法进行了分析验证.从方法理论的角度,以数值实验资料为依据,本文提出的两阶段模拟法是可信的.4 结 论针对当今流行的油粒子方法忽略油膜自身扩展过程和扩散面积计算方面的不足,本文运用经典Fay理论对其进行了改进,提出了溢油运动的两阶段模拟法.数值模拟实验结果表明,两阶段模拟法因充分考虑了溢油初始阶段的自身扩展过程,能够弥补油粒子方法的不足,符合溢油在不同时期扩散机制亦不同这一实际情况,比油粒子方法更能真实地反映溢油的运动规律.[参 考 文 献][1] REED M,J O HANSEN O,BRANDVIK P J,et al.Oil spill modeling towards t he close of t he20t h century:over2view of t he state of t he art[J].Spill Science&Technology Bulletin,1999,5(1):3216.[2] 李冰绯.海上溢油的行为和归宿数学模型基本理论与建立方法的研究[D].天津:天津大学,2004.L I B F.Study on t he basic t heory and establishing met hod of t he mat hematical modeling of oil spill on t he sea[D].Tianjin:Tianjin University,2004.[3] 王长海.溢油漂移扩散计算模式初步研究[J].交通环保,2000,21(2):729.WAN G C H.Preliminary study on spilled oil drift and diffusion calculation mode[J].Environmental Protection in Transportation,2000,21(2):729.[4] 娄安刚,王学昌,孙长青,等.胶州湾海面溢油轨迹的数值模拟[J].黄渤海海洋,2001,19(1):128.LOU A G,WAN G X C,SUN C Q,et al.Simulation of oil spill trajectory on sea surface of Jiaozhou bay[J].Jour2 nal of Oceanography of Huanghai&Bohai Seas,2001,19(1):128.[5] BLO KKER P C.Spreading and evaporation of product s on water[C]∥Proc of4t h Internal Harbour Congress.Antwerp,t he Net herlands:[s.n.],1964:9112919.[6] FA Y J A.Physical processes in t he spread of oil on a water surface[C]∥Proc Conf Prevention and Control of Oil69第3期刘伟峰,等:海上溢油运动数值模拟方法的探讨与改进79Spills.Washington DC:American Petroleum Institute,1971.[7] MACKA Y D,PA TERSON S,TRUDEL K.A mat hematical model of iol spill behavior[R].Environment CanadaReport EE27,1980.[8] L IU S L,L EENDER TES J J.A32D oil spill model wit h and wit hout ice cover[C]∥Proc of t he Internal Symposi2um on Mechanics of Oill Slicks.Paris,France:[s.n.],1981.[9] L EHR W J,CE KIR GE H M,FRA GA R J,et al.Empirical studies of t he spreading of oil spills[J].Oil and Pet2rochemical Pollution,1984(2):7212.[10] 武周虎,赵文谦.海面溢油扩展、离散和迁移组合模型[J].海洋环境科学,1992,11(3):33240.WU Z H,ZHAO W Q.A model of spreading,dispersion and advection caused by an oil slick on t he unstable sea surface[J].Marine Environmental Science,1992,11(3):33240.[11] 金梅兵.近岸溢油的全动力预测方法研究[J].海洋环境科学,1997,16(1):30236.J IN M B.Study on t he met hod of t he dynamical prediction of spill oil inshore[J].Marine Environmental Science, 1997,16(1):30236.[12] 尹宝树,刘浩.海面溢油对流扩散的反向计算[J].海洋环境科学,2004,23(2):16219.YIN B S,L IU H.Backward calculation based on t he advection and diffusion of oil spills at sea[J].Marine Envi2 ronmental Science,2004,23(2):16219.[13] J O HANSEN O.The Halten Bank experiment2observations and model studies of drift and fate of oil in t he marineenvironment[C]∥Proceedings of t he11t h Arctic Marine Oil Spill Program(AMOP)Technical Seminar.Environ2 ment Canada,1984:18236.[14] ELL IO T A J.Oceanic processes and NW European shelf databases[J].Marine Pollution Bulletin,1991,22(11):5482553.[15] 张存智,窦振兴,韩康,等.三维溢油动态预报模型[J].海洋环境科学,1997,16(1):22229.ZHAN G C Z,DOU Z X,HAN K,et al.A t hree dimensional model to predict t he behavior of oil spill[J].Marine Environmental Science,1997,16(1):22229.[16] EL HA KEEM A A,EL SHORBA GY W,CH EBBI R.Oil spill simulation and validation in t he Arabian(Persian)Gulf wit h special reference to t he UA E coast[J].Water Air Soil Pollution,2007,184:2432254.[17] CH EN H Z,L I D M,L I X.Mat hematical modeling of oil spill on t he sea and application of t he modeling in Dayabay[J].Journal of Hydrodynamics,2007,19(3):2822291.[18] WAN G S D,SH EN Y M,GUO Y K,et al.Three2dimensional numerical simulation for transport of oil spill in seas[J].Ocean Engineering,2008,35:5032510.。
多声道超声波流量计内部流-声耦合特性仿真分析

doi: 10.11857/j.issn.1674-5124.2020100129多声道超声波流量计内部流-声耦合特性仿真分析马雪林1, 徐 雅1, 谢代梁1, 徐志鹏1, 曹松晓1, 胡鹤鸣2(1. 中国计量大学 浙江省流量计量技术重点实验室 , 浙江 杭州 310018; 2. 中国计量科学研究院, 北京 100029)摘 要: 时差法超声波流量计的测量精度的提升难点在于明晰声波在其内部的传输问题。
为探究在水流量测量中多声道超声波流量计的使用及其流-声耦合特性,对一个DN200的5声道超声流量计进行流-声耦合的数值模拟研究。
利用RKDG 法求解声传播方程,定量分析不同声道截面下流速分布、换能器端面的声压信号分布,并给出不同声道下声波在管道流体中的传播动力学过程,分析声波与管壁之间的相互作用及其对信号传输的影响,得到该模型下不同流速时的系统计算误差。
该仿真分析中所涉及的流-声耦合特性研究结果对多声道超声波流量计测量性能的提升具有重要意义。
关键词: 多声道; 超声波流量计; 流声耦合; 数值模拟中图分类号: TH814; TN64文献标志码: A文章编号: 1674–5124(2021)05–0123–06Simulation analysis of flow-acoustic coupling characteristics inmultichannel ultrasonic flowmeterMA Xuelin 1, XU Ya 1, XIE Dailiang 1, XU Zhipeng 1, CAO Songxiao 1, HU Heming 2(1. Key Laboratory of Flow Measurement Technology of Zhejiang Province, China JiliangUniversity, Hangzhou 310018, China; 2. National Institute of Metrology, China, Beijing 100029, China)Abstract : The difficulty in improving the measurement accuracy of the time difference ultrasonic flowmeter is to understand the internal sound wave transmission problem. In order to explore the use of multichannel ultrasonic flowmeter in water flow measurement and its flow-acoustic coupling characteristics, a numerical simulation study of flow-acoustic coupling was carried out on a DN200 5-channel ultrasonic flowmeter. The RKDG method is used to solve the sound propagation equation, the velocity distribution on the sound channel and the sound pressure signal distribution on the end face of the transducer are quantitatively analyzed, get the dynamic process of sound wave propagation in pipe fluid under different sound channels, the interaction between acoustic wave and tube wall and its influence on signal transmission are analyzed, the system calculation error at different flow rates under this model is obtained. The research results of the flow acoustic coupling characteristics involved in the simulation analysis are of great significance to the improvement of the measurement performance of the multichannel ultrasonic flowmeter.Keywords : multichannel; ultrasonic flowmeter; flow-acoustic coupling; numerical simulation收稿日期: 2020-10-30;收到修改稿日期: 2020-11-10基金项目: 国家重点研发计划(2018YFF0216001)作者简介: 马雪林(1996-),女,宁夏固原市人,硕士研究生,专业方向为超声波流量测量。
PIV与PLIF同步测量方法在湍流扩散研究中的应用

第35卷第1期2019年2月上海电力学院学报Journal of Shanghai University of Electric PowerVol.35,No. 1Feb. 2019D O I:10.3969/j.issn.1006 -4729.2019.01.017P IV与PL IF同步测量方法在湍流扩散研究中的应用付在国,赵飞宇,张莉,刘江(上海电力学院能源与机械工程学院,上海200090)摘要:为了定量分析湍流中浓度、温度等标量的输运过程,需要同时获取流场空间内速度和标量的脉动值,以确定湍流扩散特征量。
详细介绍了同步应用粒子图像测速与平面激光诱导荧光技术开展测量和数据处理的方法,并应用该方法获取了槽道内两种流体的湍流扩散通量等特征量。
研究结果表明,该方法可有效地应用于湍流扩散研究领域。
关键词:粒子图像测速;平面激光诱导荧光;脉动速度;标量输运;湍流扩散中图分类号:〇357.5 文献标志码:A文章编号:1006 - 4729(2019)01 -0090 - 06 Application of Simultaneous PIV and PLIF Measurements inTurbulent Diffusion StudyF U Z a i g u o,Z H A O Fe i y u,Z H A NG L i,L I U Jiang(School of Ener^^y and Mechanical Engineering,Shanghai University of Electric Power,Shanghai 200090,China)A b s t r a c t:T o do quantitative analysis for the transport process of scalars in turbulence,such asconcentration and temperature,i t is necessary to acquire the velocity and scalar fluctuations value inflow fields at the s a m e time so that to determine characteristics of turbulent diffusion.T of measuring and data processing b y application of simultaneous particle image velocimetry (P I V)and plane laser-induced fluorescence (P L I F)techniques a e introduced in detail.M o r e o v e r,themettiods a e used to obtain the turbulent mas s flux of t w o fluids in the turbulent channel flow.T h eresults s h o w that the m e t h o d can be applied effectively in the field of turbulent diffusion.K e y w o r d s:particle image velocimetry;plan laser-induced fluorescence;fluctuation velocity;scalar transport;turbulent diffusion随着激光测速和激光诱导荧光技术的发展,粒子图像测速(Particle I m a g e Velocimetry,P I V)和平面激光诱导荧光(P l n e r Laser-Induced Fluorescence,P L I F)技术已广泛应用于湍流与燃烧等 场合下流动介质的速度场测试、结构显示与标量 输运测量等方面。
材料英语证书考试(PEC)-材料处理词汇
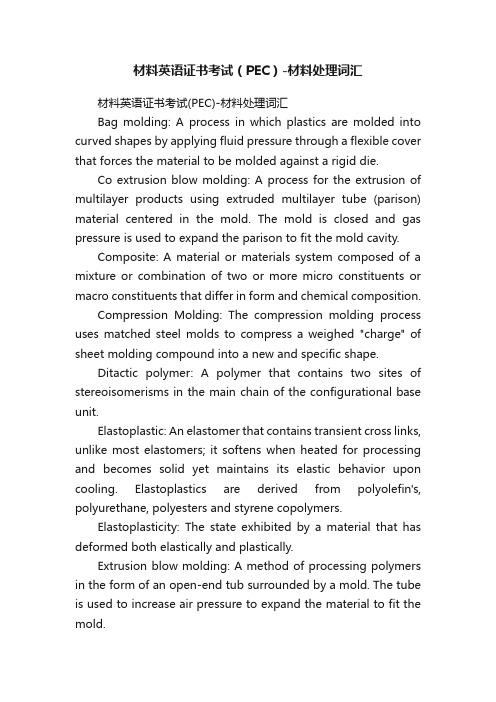
材料英语证书考试(PEC)-材料处理词汇材料英语证书考试(PEC)-材料处理词汇Bag molding: A process in which plastics are molded into curved shapes by applying fluid pressure through a flexible cover that forces the material to be molded against a rigid die.Co extrusion blow molding: A process for the extrusion of multilayer products using extruded multilayer tube (parison) material centered in the mold. The mold is closed and gas pressure is used to expand the parison to fit the mold cavity.Composite: A material or materials system composed of a mixture or combination of two or more micro constituents or macro constituents that differ in form and chemical composition.Compression Molding: The compression molding process uses matched steel molds to compress a weighed "charge" of sheet molding compound into a new and specific shape.Ditactic polymer: A polymer that contains two sites of stereoisomerisms in the main chain of the configurational base unit.Elastoplastic: An elastomer that contains transient cross links, unlike most elastomers; it softens when heated for processing and becomes solid yet maintains its elastic behavior upon cooling. Elastoplastics are derived from polyolefin's, polyurethane, polyesters and styrene copolymers.Elastoplasticity: The state exhibited by a material that has deformed both elastically and plastically.Extrusion blow molding: A method of processing polymers in the form of an open-end tub surrounded by a mold. The tube is used to increase air pressure to expand the material to fit the mold.Fiberglass: Used to reinforce and/or strengthen plastics.Hydro plasticity: The occurrence of plastic behavior.Injection molding: A process where molten plastic is injected into a mold. About 60% of all plastics processing machines are injection molding types.LPMC?: A low pressure molding compound, similar to sheet molding compound (SMC), but is moldable at pressures around 100 lbs/psi.Micro moulding: An emerging technology that takes injection molding technology down to a smaller scale.Mold 24i: is a new material-evaluation feature that uses the press as a tool to gauge melt viscosity and determine operating temperatures, pressures and velocities.Mold: A hollow form into which molten plastic is poured to form a designed shape when the material cools.Plastic anisotropy: V ariations in directions in the resistance of a material to plastic deformation.Plastic deformation: The permanent deformation arising from the relative displacement of atoms or molecules.Plastic Zone: A heavily plastically deformed region in a material, generally adjacent to the tip of a crack that can cause crack-tip blunting.Plasticity: Fact of being plastic.Plasticization: A process used to lower glass transition temperature; to improve the flexibility of certain polymers, allowing them to remain flexible well below the glass transition temperature of unplasticized materials.Elasticized: A material that is capable of imparting flexibility to nonplastic material.Plasticorder: Instrument that measures the temperature,viscosity and shear-rate relationships of a plastic substance to determine its eventual behavior.Plasticoviscosity: Material whose rate of plastic deformation when subjected to stresses exceeding the yield stress is a linear function of the applied stresses.Plastics: Materials of high molecular weight that consist primarily of synthetic polymers or condensates which can be shaped by flow into objects of diverse shapes and sizes.Plastisol: Dispersion of resin in a plasticicizer that gels when heated.Polystyrene acrylonitrile resin: A tough thermoplastic copolymer with excellent resistance to acids, banes and a few solvents.Polyurethane Foam: A flexible or rigid cellular substance that is created through the reaction of a polyester with a diisocyanate, used primarily for insulation or padding.Ram-fed injection molding: A high pressure injection molding process that utilizes a ram to force the molten plastic into a mold.Reciprocating-screw molding machine: An apparatus used in polymer processing in which pelletsof a plastic are fed, compacted, melted and then forced into a mold cavity by means of a plunger.SAAM (Self Aligning Actuating Mold): A low pressure, portable, turn-key. production molding system that requires no special site preparation.Sandwich molding: A process by which a skin core structure, typically of two different polymers, is produced in a twin injection process.Segmented polymer: Any of a class of thermoplasticelastomers derived from aromatic dicarboxylic acids, polyalkylene-ether glycols and short-chain diols; used for injection molded parts for footwear and automobiles and for wire coating, pressure sensitive adhesive, belts and hoses.SMC (Sheet Molding Compound): A fiberglass reinforced composite material produced in a "sheet" format.Specific modules: The modules of elasticity divided by the density.Spectroscope: A device that can be used to identify the chemical composite of plastic waste containers and scraps.Stress plastic: Show the stress lines in plastic using polarized light.Stretch blow molding: A thermoplastic forming process in which an extruded or injection molded parison is stretched axially at a temperature close to its softening temperature and blow, either simultaneously or in two stages, to produce a final product of desired dimensions.Styrene plastic: A plastic compound of polymerized styrene or copolymers containing styrene.Styrene acrylonitrile resin: A solid thermoplastic copolymer of styrene and acrylonitrile that resists scratching, chemicals and stress.Styrofoam: A form of polystyrene foams that is used in insulating materials, ice chests, drinking cups and flotation devices.Superplasticity: The ability of a material to deform uniformly by an exceptionally large amount, usually at elevated temperatures.Superpolymer: An extensive setting up of cross links between molecular chains, resulting in an infusible polymer suchas in a thermosetting resin.Symmetric laminates: Laminated fibrous composites with symmetrical stacking sequences for each ply above the midplane and there is a ply identical in composition and orientation at an equal distance below the midplane.Synthetic polymer: A polymer that is manmade, such as plastics, elastomers and adhesive.Thermoplastic recording: A form of recording in which the information is permanently impressed on plastic tape.Thermoplastic: A group of polymers or relating to textile fibers and resin that can be easily softened on heating at high temperature; all synthetic fibers are thermoplastic.Twin-screw compounding system: A system for mixing polymers with additives such as pigments, lubricants, stabilizers, cross-linking agents and plasticizers.Twinning deformation: A plastic deformation process in which atoms are displaced to form a mirror image of the underformed region.V acuum forming: Plastic sheet forming in which a thermoplastic sheet is clamped to a holder and then heated and drawn into the mold by a vacuum.。
畜牧专业英语词汇(养猪方向]
![畜牧专业英语词汇(养猪方向]](https://img.taocdn.com/s3/m/5a984daebceb19e8b8f6ba72.png)
一、生产控制记录Production control recordings1000 day calendar1st matingsactual vs targert atrophiedbarn recordsboars inboars outboars use chart chronological recordscomputer assisted -production controlconception conception rateculldaily activity report daily activity sheet daily gaindeath ratedressing percentear tagear tattooearly abortion femalefirst matings farrowingfarrowing control chartfarrowing register gaingestationgilts exposed not yet matedgilts ingilts to herdherd register inseminationlate term abortion litter sizemale matingmarking stickmating patternmating register千天日历首次配种数实际:指标萎缩的舍内用的记录表新进群的公猪数淘汰公猪数公猪使用(频率)图表按时间计算的记录电脑支助性生产控制受胎、受孕受胎率淘汰每日事件报告每日事件记录表日增重死亡率屠宰率耳牌耳刺早期流产雌性、母猪第一次配种数产仔产仔控制图表产仔记录增重怀孕待配青年母猪数进群青年母猪数进群青年母猪数猪群记录授精后期流产窝产头数雄性、公猪配种记号笔配种计划配种记录mortalitymummifiedparityparturitionpen cardpig identificationpig movementsregisterpiglets born alivepiglets born deadpiglets out of nurserypiglets to nurserypiglets weanedpostweaningprematurerecord cardregisterregistersreturn matingssalessow cardsow + litter cardsows not yet matedsows outsows weanedsucking pigstargettotal pigs borntotal matingsvarianceweaningweekly productioncontrol死亡率木乃伊胎次分娩猪栏卡猪只标识猪只移动记录产活仔数产死仔数转出保育舍猪数转入保育舍猪数断奶仔猪数断奶后早熟记录卡登记登记、记录返情配种数销售数母猪卡母猪卡+窝猪卡待配母猪数淘汰母猪数断奶母猪数哺乳仔猪数指标仔猪总出生数总配种数变异断奶星期(式)生产控制二、猪的内部器官Internal organsanterior pituitary appendixaortaartery backbonebile duct bladderblood vessels braincaecumcolon diaphragm duodenumfemurheart hypothalamus jejunumkidneylarge intestine lipsliverlungmouthnerves oesophagus ovaryoviduct pancreaspelvisposterior pituitary rectumseminal vesicals sensory organs shoulder blade skeletonskullsmall intestine spleenstomach teethtesticles垂体前叶阑尾大动脉动脉脊椎骨胆管膀胱血管脑盲肠结肠横膈膜十二指肠大腿骨心脏下丘脑空肠肾脏大肠唇肝肺嘴神经食道卵巢输卵管胰脏骨盆垂体后叶直肠精囊感觉器官肩胛骨骨骼头盖骨小肠脾脏胃牙齿睾丸throatthyroidtongueurethrauterusveinvertebrae喉咙甲状腺舌头尿道子宫静脉脊椎三、健康综合词汇Health terms generalacclimatizationair bornAIall in all out anthelmintic antibodyantibioticantigenappetite attenuated live vaccinebacteriabacterin bacteriology bacteriosis biosecuritybody condition blood testcasecontaminant contamination contamination zone cross transmission diagnosisdiseasedisease barrier disease control disease free disease transmission disinfectdilution theory embryo transfer frozen semen healthhealth control health control programhealth control system health insurancehealth profilehealth statushygienehysterectomy驯化适应空气生成、空气产生人工授精全进全出驱蠕虫药抗体抗生素、抗菌素抗原食欲弱毒疫苗细菌灭活菌苗细菌学细菌病生物安全躯体状况血液检验病例、病症污染物污染污染带、污染区交叉传染诊断疾病疾病屏障疾病控制无病疾病传播消毒稀释原理胚胎移植冷冻精液健康健康控制健康控制方案健康控制体系健康保险健康概况健康状况卫生子宫切除术immune systeminjectionisoweanisolationmanageable disease riskmedicated earlyweannew born baby pigtransfernose to noseon-farm health programprofilequarantinespread of diseasestocking densitysymptomvaccinevaccinationvectorsveterinarianveterinary medicinevirus免疫系统、免疫体系注射药物早期断奶隔离能把握的疾病风险药物早期断奶新生仔猪转移鼻对鼻(疾病传播)场内健康方案轮廓、概况检疫疾病传播圈养密度状况疫苗注射疫苗病菌媒介兽医兽医学病毒四、猪的身体和形体Part of pig and conformationanusbackbackfatbellycervixcheek conformation crown(of ?)dew clawdipbackearseyemuscle eyemuscle area eyesexternal reproduction organsfacefeet/footfore flankforeleggaithamhairhiphockhoofjowlknucklelengthloinmid back line narrow chestnecknosepadpasternpenis pollrear legrear flankrumpscrotum肛门背部背膘腹部子宫颈脸颊体形、形体蹄冠悬蹄、假蹄凹背耳朵眼肌、背最长肌眼肌面积眼睛外生殖器脸、面部足前胁前腿步态大腿、臀部毛屁股跗关节蹄下颚膝关节长度腰背中线狭窄胸部颈部鼻子蹄垫系部、第一指(趾)关节阴茎头顶后腿后胁尾部、臀部阴囊shouldersheathsickle hocksideskinskin conditionsnoutsway backedtailtailheadteats/nipplestesticlestip toetoenailstoesturbinatestusks(of boar)udderumbilical cordunderlineuneven claws(toes)vulva肩部包皮撇腿体侧皮肤皮肤状况猪嘴(鼻)斜背尾巴尾梢乳头睾丸脚趾头脚趾甲趾鼻甲软骨(公猪)犬齿乳房脐带乳房不齐趾阴户五、生产系统、建筑Production system and buildingsaggregateair flow, air speed air pockets angle ironcrate\stall asbestosbird screenblack pipeblue printsboar penboar stallB.O.Dbowl drinker brickbrick wall building design castiron slat ceiling\attic cementcement block cement skim centre to centre clampcomfort\feed tray comfort mat comfort zone concrete concrete slat cross section curtain material curtain rod curtain-sided designdoordormitory drinker bracket dripperelevationend to endend weightexpanded bolt瓜米石空气流动,气流速度气域、气团角铁定位栏石棉瓦防鸟网黑管蓝图公猪栏公猪定位栏生物耗氧量碗式饮水器砖砖墙建筑设计铸铁漏缝天花板水泥水泥砖水泥面中对中夹子舒适/饲料垫舒适垫舒适地带混凝土水泥漏缝截面卷帘材料卷帘杆边墙卷帘设计门宿舍饮水器架滴水装置上升,提高边对边终测体重膨胀螺栓eye bolt\hookfan\variable speedfanfarrowing cratefarrowing housefarrow to feeder pigfeedmill\storefinger barfinisherflat steelfloor profileflushfootingsgategate hingegate latchgestationgroup pengrow-outgaivanized pipegutterheatlamphot dip galvanizedindividual stallinfrastructureinside to insideinspection holeinsulated roofinsulationlevelliquid manurelow spotsmanure guttermanure handlingmanure lagoonmanure pitmating areamating penmetal sheetingmetal slatmicro-environmentmister风钩风扇/变速风扇分娩栏、产床分娩舍分娩至保育猪饲料厂/仓库耙齿育肥舍扁钢地面形状冲洗地基栏门门链门闩妊娠舍群体栏场外育肥舍镀锌管排粪沟产热灯热镀锌(表面)单体限位栏基础设施内对内检查孔绝缘屋顶绝缘水平稀粪低点粪沟粪便处理化粪池粪坑配种区域配种栏金属板金属漏缝条微环境雾化器moveable side curtainmultisitemultilayer floor nipple drinker nonslip finish nurserynursery deck nursery feeder offsetoff-teston-testopen flushopen gutter flush outside to outside overhangpen dividerpen wallperimeter wallpierspig buildingpiglet floorpit plastic coated metalplastic slatpollutionprocessing/storagepull the plugpulleyrelative humidityraised farrowingdeckrebarreduction gearroof panelroof pitch\sloperoof tileroof ventsroperump barsandsick penside panelside to sideside wallsite layout活动边窗卷帘多场式多次成形水泥地面鸭嘴饮水器不滑的育肥舍保育舍保育床保育猪食箱独立饲养区结束测试开始测试无漏缝盖板地沟无漏缝盖板地沟外对外外檐隔栏栏墙围墙柱子猪舍仔猪地面坑塑料包金属塑料漏缝污染加工/贮存拉开塞子滑轮相对湿度高架分娩床螺纹钢齿轮屋面瓦屋顶斜度瓦屋顶通风绳子后部挡棍沙病猪栏边栏板边对边边墙场地平面规划single pour concretefloorsingle sitesingle stageslat ledgeslopedslot/slat ratiosmooth rodsolid manuresolid rodsow feedersow floorsow stallspray bake paintsprinklersteelstep downswinging drinkersupplementary heatT bolttemperaturethermostattroughtrusstwo stagetop bartop beamU boltunderground manurepipelineventilationverandahvertical barsvibratorwater/elect/roadsweldwinch/crankwindowswire rope(cable)woven wire floor一次成形水泥地面一个场址一个阶段漏缝板撑架坡度漏缝与漏缝条之比例圆钢干粪实心钢母猪食槽母猪地面母猪定位栏喷涂、烘喷漆喷水器钢台阶摇晃式饮水器辅热器T型螺栓温度自动调温器食槽梁二个阶段上部挡棍上梁U型螺栓地下粪便排送管空气流通室外有顶走道垂直挡棍振动器水/电/路焊接摇把窗钢丝绳编织网地板六、综合词汇General termsacclimatization area acidage segregation agitationAI Catheteralkalianoestrusappetiteartificial insemination artificial service awkward sowbaby pig(piglet) baby pig scours back pressure barrow(castrated mate)bleachbloody scoursboar sow ratioboar stud breedingbreeding herd castratecervixcharactercollect(semen) colostrumcomfort zone commercial herd common environment conceivecondition continuous flowconsistencycontinuousproductioncull sowdefacatedilutiondiseasedisinfectantdummy sowearly embryonicmortality驯化适应地点酸年龄区分搅拌人工授精管碱不发情食欲人工授精人工授精、人工配种异常母猪仔猪仔猪拉稀压背测情去势公猪漂白剂血痢公母比例人工授精公猪舍育种、配种、繁殖种猪群阉割子宫颈部性状、特征采集(精液)初乳舒适带商品群、父母代群同等环境怀胎体况持续周转状况均衡生产淘汰母猪排粪稀释疾病消毒剂假母猪早期胚胎死亡率earmark/tattooear tagE. coli scoursembryore-absorptionenergyestrusexposed giltextenderfarrowfarrowing cyclefarrow to finishfarrow to weanfeedfeed grade A/Bfeed intakefinishing pigfirst servicefresh semenfrozen semengestation periodgood matingcharactergrower/finishergrowing giltgrowing pighard udderheat(oestrus)hygeinehypodermic syringeindentificationinitial populationinjectableinjectioniron injectionisolationintegrated productionsystemintensive pig farmintensive pig productionkeen boarlate term abortionlazy boarlibido耳记/耳刺耳牌黄白痢胚胎吸收能量发情待配青年母猪(精液)稀释液产仔产仔周期产下至出栏产下至断奶饲料饲料等级抗菌素采食量育肥(成)猪首次配种新鲜精液冷冻精液怀孕期良好配种特性中/大猪(生长/育肥猪)中后背母猪中猪乳头发情卫生皮下注射器识别、确认初始群可注射的注射注射铁剂隔离完整的生产体系集约化养猪场集约化生猪生产性能好的公猪晚期流产懒公猪性欲limelitterlitters/year location looseness manure, dung, shit market pigmate/mating mating areamating penmilking abilityM. M. Amodern intensiveproductionmotheringcharacteristicsmummifiedmultiple-sitemultipliernatural matingneedlenew born pigletnewly weaned sownippleno heat(anoestrus)nursing sowoestrus cycleovaovaryoviductparitypenispigpiglet creep areapiglet processpiglet born deadpigs/sow/yearpigs weaned/litterpregnancypregnantpregnancy diagnosispressure washprolificacyreplacementsreproductiveproblemscalpel石灰窝(猪)年均产仔窝数地理位置软粪粪便上市商品猪配种配种地点配种栏泌乳能力产后无乳综合症现代集约化生产母性特征木乃伊多场址繁殖场自然交配注射用针头新生仔猪新近断奶母猪乳头不发情哺乳母猪发情周期卵子卵巢输卵管胎次阴茎猪仔猪补料区仔猪处理死产仔猪母猪年均出栏生猪窝均断奶仔猪妊娠妊娠妊娠诊断高压冲洗多产性更新繁殖障碍解剖刀scourseasonal productionselected giltsemensigns of heatsiteslaughterslaughter housesowsow performancesplit sex feedingstimulatesucking pigletsucklesupervised matingtesticlesthree-siteT. N. T(teeth-navel-tail)traditionalproductiontwo-siteurinevaginavaccinatevaccination programvesectomizedboar(chaser)vomit, regurgitatevulveweanweaned pigweaned sowweaning ageweekly production痢粪季节性生产选作种用的小母猪精液发情征兆场址屠宰屠宰场母猪母猪性能分性别饲喂刺激哺乳仔猪哺乳监助配种睾丸三个场址齿-脐-尾传统性生产二个场址尿阴道注射疫苗免疫程序经结扎的公猪呕吐阴道断奶断奶仔猪断奶母猪断奶日龄按周生产(星期生产)七、小工具、工具和必需品Small equipment, tools and suppliesadjustable wrench AI catheter barrowboltbowl drinker broombucketcement trowel chaincrowbar disinfectant bath drilldrill bitdrippeeartag pliersear notchers electric prodfeed cartfeed scoop flexible hose forcepshacksaw hacksaw blade hammerhoehole punchers hose clamp hypodermic needle in line medicator lean meter light switchmarking stickmistermultidose syringenailneedle+suturenipple drinkernutP. D. Machinepen(for pig)pen(for writing)活动扳手人工授精管手推车螺栓,螺钉碗式自动饮水器扫帚水桶涂水泥的铲子链条,链子铁撬,铁棍消毒浴锥子,钻孔机钻头滴水器耳牌钳耳缺钳电棍运料车舀料勺软橡皮管镊子弓锯弓锯刀(锯条)铁锤,榔头锄头打(耳)孔器软管夹钳注射器针头自动饮水加药器背膘测定仪灯开关记号笔喷雾器连续性注射器钉子缝合针+缝合线饮水器螺母,螺帽怀孕探测仪猪栏笔pig boardpig catcher(snare)piglet cartpiglet pullerpliersplungerpressure hosepressure washerquick couplersaw(wood)scalpel+bladescissorsscrewscrewdrivershovelspannerspringsprinklersterilizersyringetag marking pentape measuretail cuttertail cutterizertattoo digits+nos.teeth clippersthermometerultrasonicvicegripswasherwater pipewater tapwater valveweigh scale转猪板保定器仔猪运送车助产绳钳子塞子耐压橡皮管高压冲洗机碰珠式嵌头木棍手术刀柄+刀片剪刀螺丝,螺栓螺丝刀铁锹,铲扳手弹簧洒水器蒸煮消毒器注射器耳牌笔卷尺断尾钳电热断尾钳耳刺上的数字+字母剪牙钳温度计,体温计超声波线头固定钳垫圈水管水龙头水阀秤八、遗传、品种改良Genetic , breeding improvementacceleration stage additiveatavism(reversion) backcrossbackfat depth carcass quality carcass yield percent character/traitC. + K. + L.close breeding closed herd commercial commercial breeding valueconformation characteristics contemporary comparison corrected D.O.T. correlation crossbreedingcross combination cruising stage Berkshiredaily live weight gain damdamlineDanishDallanddays on test Dekalbdisease resistancedissected leanDuroceconomic valueefficiencyend of testestimated breedingvalueenvironmentEuribridF1 crossbredfeed conversionfeed efficiencyfitness加速期、加速阶段加性、累加、添加返祖遗传、隔代遗传返交、回交背膘厚度胴体质量胴体出肉率特性CKL背膘近交封闭式猪群商品群经济育种值外形特征同期比较经校正的测验天数相互关系杂交、杂交育种杂交组合维持期、平稳阶段巴克夏每日活增重母系、雌性母系品系丹麦的国外某种猪公司名称测试期天数迪卡白公司及猪品种抗病力分解瘦肉杜洛克猪经济价值效益测验结束估测育种值环境国外某种猪公司名称杂交一代饲料转换率饲料转换率适合性、适度性generation intervalgeneration turn overgenetic correlationgenetic defectgenetic engineeringgenetic potentialgenetic progressgenetic statusgenetic valuegenotype (G)(G): (E) interactionG. G. P.G. P.group testinggrowth curvegrowth efficiencygrowth ratehalothane testHampshirehealth statusheritableheritabilityhybrid vigorinbreedinginbreedingcoefficientindividual testingintake(feed)inter-muscular fatintra- muscular fatLandraceLarge whitelean:fat ratiolean percentageWORD完美格式lean tissue F.C.R. line breedinglocal breeds loineye area loineye depth measurablemeat quality Meishan世代间隔世代周转遗传相关遗传缺陷遗传工程学遗传潜力遗传进展、遗传进度遗传现状遗传值、育种值遗传种类(类型)G.和E.的相互影响性曾祖代祖代群体测试生长曲线生长率生长率一种检验应激基因的试验汉普夏猪健康现状可遗传的、遗传的遗传力、遗传率杂种优势、杂交优势近交近交系数个体测试(饲料)摄取量肌间脂肪肌内脂肪兰德瑞斯、长白猪大白猪、大约克猪瘦肉和肥肉的比例瘦肉率瘦肉组织饲料转换率品系配种、品系繁育地方品种眼肌面积眼肌厚度可测(衡)量的肉质梅山猪migrationmultipliermuscle qualitymutationnonadditivenucleusP.pedigreeperformance testingphenotypePICPietrainpig born/litterprobabilityprogeny testingprolificacyP.S.I.purebreedingpure line/breedqualitive geneticsrandom breedingrealtime u/sreproductiveSeeghurselection indexselection intensityselection pressuresiresirelinesow productivitystandard deviationstart of teststresssubcutaneous fattesttotal body fatultrasonicvariationvisual appraisalwatery porkwestern breeds(基因)转移、迁移繁殖(群)肉质突变不累加核心(群)父母代系谱性能测试混合型PIC猪种改良公司皮特兰猪窝产仔数概率、可能性后代测试多产性、多胎性、增殖力应激白肌(猪)肉纯种繁育、纯配纯种、原种质量遗传学随机配种繁殖的斯格猪选择指数选择强度选择强度父系、雄性父系品系母猪繁殖力、生产力标准偏差开始测试应激皮下脂肪测试身体脂肪总量超声波变异肉眼评定、体型鉴定含水猪肉西方品种WORD完美格式九、抗菌素、激素、药品类Antibiotics and drugsANTIBIOTICS albipenampicillin(-S) aureomycin basitracin(BMD) baytril chloramphenicol chlorotetracycline chlorotetracycline calciumdepocillin depomycin doxycyclin duplocillin engemycin 10% enrofloxacin furacillin furazolidon gentomycin kanamycin neoditorneomycin sulfate norfloxacin norfloxacin(powder) oxybiocycline oxytetracycline oxytetracycline calcium penicillin streptomycinsulfa-drugsterramycintetracyclintiamulintribrissentylantylosintylosin injectiontylosin phosphatepremixtylosin tartrat solublepowder抗菌素安必清氨苄青霉素(钠)金霉素杆菌肽锌百病消氯霉素金霉素、氯四环素金霉素钙盐普鲁卡因青霉素普鲁卡因青链霉素强力霉素(脱氧土霉素)长效青霉素安净霉素10%恩诺沙星呋喃西林痢特灵(呋喃唑酮)庆大霉素卡那霉素止泻灵硫酸新霉素烟酸诺氟沙星氟哌酸烟酸盐(原粉)盐酸四环素土霉素土霉素钙盐青霉素链霉素磺胺类药土霉素四环素泰妙菌素畜幼生泰乐菌素泰乐菌素泰乐菌素注射液10%磷酸泰乐菌素预混剂酒石酸泰乐菌素可溶粉HORMONEoxytocinPG-600pituitrinprostaglandinsulpart / prostaparsuprareninintertocine-SOTHER DRUGSalbendazoleallocaineamidazonanalginatropineatropine sulfatechloropromazineformalinglucose salinelvomeclactasinumneguvonPBH Spraypiglet lronprocainesaccharomycessiccumSOA Spray sodium chloride 激素催产素催情素后叶激素前列腺素其他药物阿托品硫酸阿托品氯丙嗪福尔马林葡萄糖生理盐水普鲁卡因生理盐水十、营养、饲料Nutrition and FeedingINGREDIENTS alfalfa meal barleybone meal blood mealcorn cottonseed cake dicaldried whey 70% fishmealfull fat bean limestonemeat+bone meal micromineral pac milk powder oatsoilplasma protein pop bean rapemealricerice branrice hullssalt sesameseed cake sorghum(milo) soybean meal sunflowerseed mealtapioca-maniocvitamin pacwheatwheat branwheat midswhey powderyeastNUTRIENTSamino acidsantimoldantioxidant饲料原料苜蓿草粉大麦骨粉血粉玉米棉籽饼磷酸氢钙70%乳清粉鱼粉膨化大豆石灰粉、碳酸钙、壳粉肉骨粉复合微量元素全脂奶粉燕麦油脂血浆蛋白膨化大豆菜籽饼稻谷、大米米糠粗糠盐芝麻饼高粱豆粕葵花籽饼地瓜、红薯复合维生素小麦麦麸、麸皮四号粉(次粉)乳清粉酵母(菌)营养物质氨基酸防霉剂抗氧化剂arginineavailable phosphorus4% basemixbiotincalciumcholinechromiumcobaltconcentratecoppercrude fibercrude proteincyanocobalamin B12cysteineessential amino acidsfolacinglucosehistidineiodineironisoleucinelactoseleucinelysinemacromineralsmagnesiummanganese menadione methionine niacin pantothenate B3 phenylalanine phosphorus 1% premix protein pyridoxine B6 riboflavin B2 selenium starch thiamine threonine精氨酸有效磷4%预混料生物素钙胆碱铬钴料精铜粗纤维粗蛋白维生素B12胱氨酸必需氨基酸叶酸葡萄糖组氨酸碘铁异亮氨酸乳糖亮氨酸赖氨酸常量元素镁锰甲萘醌(Vk)蛋氨酸尼克酸维生素B3苯丙氨酸磷1%预混剂蛋白质维生素B6维生素B2硒淀粉维生素B1苏氨酸tryptophanvalinevitaminszincFEED & FEED MILLaugerbucket elevatorautomatic feedingautomatic switchbaggerbagsewing machineblend/mixturecomplete feedconcentratecreep feeddigestible energydown spoutenergy (GE)feed additivesfeed augerfeedbag tagfeed binfeed formulafeed grade antibioticfeed millfeed supplementfinisherflavoringgilt developergrindergross energygrower feedgrowth stimulanthammer millhand-addhorizontal mixerinert materialingredient binlactation feedmealmetabolizableenergymicrominerals色氨酸缬氨酸维生素类锌饲料和饲料厂螺旋输送机提升机自动饲喂自动开关灌包机缝包机混合全价饲料精料乳猪料消化能(DE)出料口能量饲料添加剂饲料绞龙输送机料袋标签(散装)饲料仓饲料配方饲料级抗菌素饲料加工厂外加饲料大猪料食用香料后备母猪料粉碎机总能生长猪料促生长剂粉碎机手工加料混合机惰性原料原料仓哺乳母猪料粉料代谢能(ME)微量元素moisture contentmold contaminationnet energynursery feedparticle sizepelletpelletterphase feedingpiglet feedpremix recipeseparationturn headvertical mixerweevil/beetleweigh scale水分含量霉变程度净能保育猪料颗粒细度颗粒料制粒机阶段饲喂小猪料预混料配方均匀度转头搅拌机饲料昆虫磅秤十一、疾病DiseaseVIRUSF.M.Dfoot & mouth disease hog choleraJap. encephalitis B. parvovirusP.E.Dpig poxporcine epidemic diarrhea pseudorabiesP.R.R.Srabiesrotovirusswine feverswine influenza swine vesicular dis. S.M.E.D.IT.G.EBACTERIAanthraxA.P.PAct. Pleuropneumonia atrophic rhinitisbordetellosisbrucellosischlamydiaclostridial scoursE. coliE. coli scourserysipelas(diamond disease)greasy pighaemophilus parasuisileitisleptospirosismiddle ear infectionM.M.Amycoplasmalpneumoniaoedema diseasepasteurellapneumonia病毒性疾病口蹄疫口蹄疫猪瘟日本乙型脑炎细小病毒流行性腹泻猪痘流行性腹泻伪狂犬病蓝耳病狂犬病轮状病毒猪瘟猪病毒性流感猪水泡病、一号病母猪繁殖障碍综合症猪传染性胃肠炎细菌性疾病炭疽病胸膜肺炎胸膜肺炎萎缩性鼻炎波氏杆菌布氏杆菌(猪)衣原体梭菌腹泻大肠杆菌大肠杆菌性拉稀(黄白痢)猪丹毒猪油脂病(皮炎)嗜血杆菌性胸膜肺炎回肠炎钩端螺旋体中耳炎产后无乳综合症支原体肺炎(喘气病)水肿病巴氏杆菌肺炎pasteurellosissalmonella enteritisdddswine dysenteryPARASITEascarid internalwormcoccidiosishyostrongylusinternal wormparasitesmangemange mitemetastrongylusoestophagumparasitestephanurus strongyloidestrichuris GENETICcleft palate deformed legs hermaphroditeno anuspitiriosis rosea scrotal rupture splay legs umbilical rupture NUTRITIONAL broken hip/back gastric ulcer goose-step parakeratosis piglet anaemia ricketssalt poisoning vitamin deficiency (猪)肺疫沙门氏菌肠炎链球菌性脑炎猪痢疾、猪血痢寄生虫病蛔虫球虫病红园线虫体内寄生虫兽疥癣疥螨肺丝虫病食道口线虫寄生虫冠尾线虫类园线虫鞭虫病先天性缺陷唇裂畸形腿阴阳猪无肛门阴囊疝(腹股沟管疝)扒腿(肢无力)脐疝营养性疾病脊椎损伤胃卡他关节僵硬病(硒缺乏)角化不全(锌缺乏)仔猪贫血骨质疏松(软骨病)食盐中毒维生素缺乏症OTHERSelectrocutionfliesrectal prolapseside-bitingstresssunburnswollen eartickstail bitingvaginal prolapsemosquitoesmycotoxin poisoningSYMPTOMSabscessconstipationswollen jointstear stained eyestwisted snoutstiff leggedshiveringlameulcercough其它触电苍蝇脱肛背侧咬伤应激中暑耳血肿虱子咬尾阴道脱出蚊子霉菌中毒症状脓肿便秘关节水肿泪屎眼歪鼻子僵直腿跛脚溃疡咳嗽。
comsol紊流模型简易课程
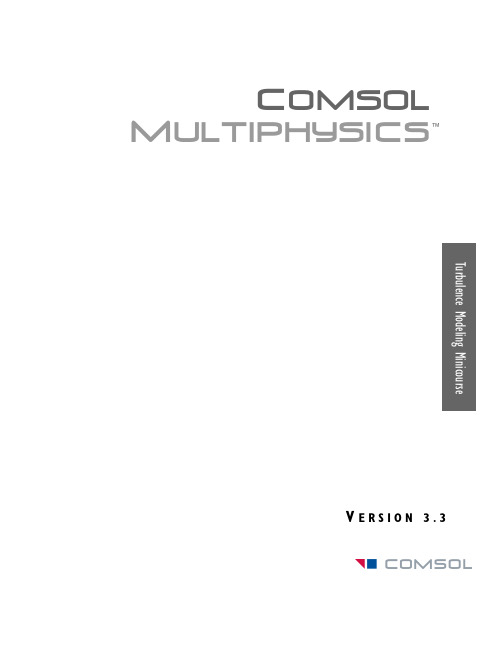
C OMSOL M ultiphysics™V E R S I O N3.3How to contact COMSOL: BeneluxCOMSOL BVRöntgenlaan 192719 DX ZoetermeerThe NetherlandsPhone: +31 (0) 79 363 4230 Fax: +31 (0) 79 361 4212 info@femlab.nlwww.femlab.nlDenmarkCOMSOL A/SDiplomvej 3762800 Kgs. Lyngby Phone: +45 88 70 82 00 Fax: +45 88 70 80 90info@comsol.dksol.dkFinlandCOMSOL OY Lauttasaarentie 52FIN-00200 Helsinki Phone: +358 9 2510 400 Fax: +358 9 2510 4010info@comsol.fisol.fiFranceCOMSOL FranceWTC, 5 pl. Robert Schuman F-38000 Grenoble Phone: +33 (0)4 76 46 49 01 Fax: +33 (0)4 76 46 07 42 info@comsol.frsol.fr GermanyFEMLAB GmbHBerliner Str. 4D-37073 GöttingenPhone: +49-551-99721-0Fax: +49-551-99721-29info@femlab.dewww.femlab.deItalyCOMSOL S.r.l.Via Vittorio Emanuele II, 2225122 BresciaPhone: +39-030-3793800Fax: +39-030-3793899info.it@NorwayCOMSOL ASSøndre gate 7NO-7485 TrondheimPhone: +47 73 84 24 00Fax: +47 73 84 24 01info@comsol.nosol.noSwedenCOMSOL ABTegnérgatan 23SE-111 40 StockholmPhone: +46 8 412 95 00Fax: +46 8 412 95 10info@comsol.sesol.seSwitzerlandFEMLAB GmbHTechnoparkstrasse 1CH-8005 ZürichPhone: +41 (0)44 445 2140Fax: +41 (0)44 445 2141info@femlab.chwww.femlab.chUnited KingdomCOMSOL Ltd.Studio G8 Shepherds BuildingRockley RoadLondon W14 0DAPhone:+44-(0)-20 7348 9000Fax: +44-(0)-20 7348 9020@United StatesCOMSOL, Inc.1 New England Executive ParkSuite 350Burlington, MA 01803Phone: +1-781-273-3322Fax: +1-781-273-6603COMSOL, Inc.1100 Glendon Avenue, 17th FloorLos Angeles, CA 90024Phone: +1-310-689-7250Fax: +1-310-689-7527COMSOL, Inc.744 Cowper StreetPalo Alto, CA 94301Phone: +1-650-324-9935Fax: +1-650-324-9936info@For a complete list of internationalrepresentatives, visit/contactCompany home pageCOMSOL user forums/support/forumsCOMSOL Multiphysics. Turbulence Modeling Minicourse.© COPYRIGHT 1994–2006 by COMSOL AB. All rights reservedPatent pendingThe software described in this document is furnished under a license agreement. The software may be used or copied only under the terms of the license agreement. No part of this manual may be photocopied or reproduced in any form without prior written consent from COMSOL AB.COMSOL, COMSOL Multiphysics, and COMSOL Script are trademarks of COMSOL AB.Other product or brand names are trademarks or registered trademarks of their respective holders. Version:September 2006 COMSOL 3.3C O N T E N T S2IntroductionAbout T urbulence Equations 3Wall Functions. . . . . . . . . . . . . . . . . . . . . . . . 4Residence Time in a T urbulent Reactor 5Introduction . . . . . . . . . . . . . . . . . . . . . . . . 5Key Instructive Elements . . . . . . . . . . . . . . . . . . . . 6Modeling Strategy . . . . . . . . . . . . . . . . . . . . . . 6The Reynolds Number. . . . . . . . . . . . . . . . . . . . . 7Modeling the Flow Using Incompressible Navier-Stokes . . . . . . . . 8Applying a Simple T urbulence Model . . . . . . . . . . . . . . . 12Parametric Study of the Eddy Viscosity . . . . . . . . . . . . . . 13Stabilization T echniques . . . . . . . . . . . . . . . . . . . . 18Mesh Convergence Analysis. . . . . . . . . . . . . . . . . . . 22The k-ε T urbulence Model . . . . . . . . . . . . . . . . . . . 23Mass Transport of T racer Species: Convection and Diffusion . . . . . . 28A Shell-and-Tube Heat Exchanger 36Introduction . . . . . . . . . . . . . . . . . . . . . . . . 36Model Definition . . . . . . . . . . . . . . . . . . . . . . . 38Results for the Flow/Heat Model. . . . . . . . . . . . . . . . . 41Modeling in COMSOL Multiphysics . . . . . . . . . . . . . . . . 41References . . . . . . . . . . . . . . . . . . . . . . . . . 49C O N T E N T S| iii |C O N T E N T ST u r b u l e n c e M o d e l i n g M i n i c o u r s eT U R B U L E N C E M O D E L I N G M I N I C O U R S E|1IntroductionThe concept of turbulence does not lend itself to a 1-page explanation. Actually,scientists have not yet reached an agreement on what exactly turbulence is and thedefinition keeps changing as we learn more about the phenomenon. Without beingtoo specific, turbulence can be said to be the chaotic motion that appears in a flowwhen the fluid is moving fast or when an object moves with high speed through thefluid.When it comes to simulating turbulence, the problem is that the turbulent motions areextremely small, often in the range between one micrometer and one millimeter.Compare this to industrial applications where the domains of interest are typical oforder meters or even bigger, and it is easy to realize that there is no possibility toresolve the turbulence.The way to circumvent the severe resolution constraints is with turbulence modeling.There are several approaches of which the so-called RANS methods are by far the mostcommon. COMSOL Multiphysics has implemented the standard k-ε model, whichdescribes the transport of the turbulent kinetic energy, k, and the rate of dissipation ofturbulent kinetic energy, ε.The use of the k-ε turbulence model reduces the resolutionrequirements by up to a factor 100 in each space direction.The flow field in itself is seldom the quantity of interest, but the primary problem ismore often, for example, the transport of some scalar quantity such as chemical speciesor heat. This minicourse gives examples of how COMSOL Multiphysics can easilyprovide a 2-way coupling between heat transfer and turbulence modeling, as well asmass transfer and turbulence.• A first model describes a baffled water purification reactor in which we investigatethe flow properties from laminar to turbulent flow.• A second model shows how to model turbulent heat transfer in a shell-and-tube heatexchanger.These are merely clean-cut exercise examples. But your modeling will not end here—you can couple simultaneous heat, mass, momentum transport, and other physics suchas magnetic and electric fields. You imagination is the only limit!Enjoy your modeling!The COMSOL Team2|TU R B U L E N C E M O D E L I N G M I N I C O U R S ET U R B U L E N C E M O D E L I N G M I N I C O U R S E |3About Turbulence EquationsAll fluid motion is described by the Navier-Stokes equations:(1)where N is the vectorial functional describing continuity in momentum and heat, and.The basis of all turbulence modeling is to apply a filter operator to Equation 1:(2)If this is a time filter, the resulting equations are known as the Reynolds Averaged Navier-Stokes equations (the RANS equations). The idea is to solve for the filtered variables . However, the Navier-Stokes equations are nonlinear, and therefore when the filter operator is “moved onto” q , extra terms appear in Equation 2:(3)where represent the turbulent effects. As can be seen, the turbulence depends on both the filtered and unfiltered, unknown variables. For example, the extra terms in the momentum-transport equations are called transport of Reynolds stresses and can, for an incompressible flow, be written as. (4)Most models of Equation 4 are of the form(5)where k is the turbulent kinetic energy and I is the identity matrix. Further, is the turbulent dynamic viscosity, which in the model is approximated bywhere ε is the rate of dissipation of turbulent kinetic energy, and is a constant.N q ()0=q ρu v w T []T =N q ()0=q N q ()R q q ,()+0=R q q ,()ρx j ∂∂–u i u ju i u j –()ηt ∇u ∇u ()T +()23--I k –ηt k ε–ηt C µk 2ε-----=C µ4 | TU R B U L E N C E M O D E L I N G M I N I C O U R S ESo far the modeling has produced two new unknown quantities. By manipulation of the momentum-transport equations, new variants for the transport of k and ε can be derived. Different approximations in these equations lead to different models, and COMSOL Multiphysics has implemented the standard by Launder and Spalding (Ref. 1).The turbulent terms of the heat-transport equation are treated in a similar manner. The continuity equation has no turbulent terms. For more details on turbulence modeling see Ref. 2.The general idea with the modeling in such situations is to let the modeled terms account for the smallest turbulent scales so as to relax the resolution requirements. At walls, however, the assumptions used to derive Equation 5 are no longer valid. If no special measures are taken, a vast number of grid cells are needed at the wall to resolve the turbulence there. The way to circumvent this problem is to apply wall functions (see Ref. 3). Here, a special boundary condition prescribes a logarithmic dependence of the variables on the distance from the wall. These special boundary conditions are therefore also known as “logarithmic wall functions”.Applying wall functions, of course, has a negative impact on the accuracy of the results compared with resolving the turbulence at the wall. But the number of computational cells saved is so great that it often make the difference between being able to complete a simulation or not.In the following exercises you will learn how to practice turbulence modeling using the prepared application modes of COMSOL Multiphysics.k ε–k ε–Residence Time in a TurbulentReactorBaffled reactors are common in the process industry for systems containing relativelyslow reactions. They make use of a baffled geometry to avoid “short-circuiting” of theinlet and outlet streams. At the same time, the baffled geometry elongates the path forthe flow stream through the reactor without making it necessary to build long, thinreactors. These type of reactors must be designed carefully as too many baffles cancreate unnecessary turbulence and pressure losses.The distribution of residence time is a useful measure of the turbulent mixing in suchreactors. It is possible to measure the maximum, minimum, and average time that atracer element takes to travel through a reactor to quantify the extent of mixing.This model studies the residence time distribution in a turbulent-baffled reactor withan aqueous solution entering the inlet at 0.1 m s-1. Assume that you can describe themodel geometry in 2D. In order to compute the residence-time distribution, thisexample first models the flow field in the reactor assuming a steady flow. In a secondstep, it uses the computed flow field to model the time-dependent material balance fora tracer species as that species enters and leaves the reactor, see Figure1. The tracer isintroduced as an inlet pulse in concentration, while the transient of the concentrationat the outlet supplies information about the residence-time distribution in the reactor.30s45sFigure 1: Snapshots of the concentration field of a tracer at different time steps as it travelsthrough a baffled reactor.T U R B U L E N C E M O D E L I N G M I N I C O U R S E|5This modeling exercise includes the following key elements:•Solving a flow field for high flow rates, which means high Reynolds number andturbulence.•Studying the effects of the Reynolds number on the flow-model’s convergence.•Setting up a simple turbulence model based on the Incompressible Navier-Stokesequations.•Studying the benefits of using streamline Petrov-Galerkin artificial diffusion forproblems with large cell Reynolds number.•Using the k-ε Turbulence Model application mode to solve turbulent flowproblems.•Adding eddy diffusivity to the material-balance equations in the Convection andDiffusion application mode.•Postprocessing to monitor the tracer concentration at the outlet as a function oftime.The residence time distribution in the reactor is determined almost entirely by the flowfield in the reactor. When flow is turbulent, then the eddy diffusivity is several ordersof magnitude larger than the molecular diffusivity so that the flow field determinesboth the tracer’s convective and diffusive transport. This example therefore focusesprimarily on the solution of the flow field using these steps:1First use the Reynolds number to estimate the nature of the flow (prior to solving).2Try to solve the model using the Incompressible Navier-Stokes application mode.Investigate if this method is reasonable to determine a meaningful output, eventhough you expect the flow to be turbulent.3To simulate turbulence, you can solve the flow field using a high value of theviscosity, representing eddy viscosity (instead of using the molecular viscosity). Youcan do this using the parametric solver, starting from a very large viscosity andgradually decreasing it until the solution fails to converge.4Compare the results from step 3 with the results obtained using a theoreticalestimate of the eddy viscosity based on inlet velocity and geometrical length scale. 6|TU R B U L E N C E M O D E L I N G M I N I C O U R S ET U R B U L E N C E M O D E L I N G M I N I C O U R S E |75The large cell Reynolds number shows that there is a risk for solution instabilities leading to poor accuracy in the results. You can use Petrov-Galerkin streamline diffusion as a stabilization method to try to improve this accuracy.6Solve the model on a refined mesh to further validate the results.7Next try using the more elaborate k -ε Turbulence Model application mode. This allows you to compare the findings to those from the simpler model in step 6.8Finally, add and solve the transient mass balance equations. The turbulent flow field from the k -ε turbulence model serves as the input to the Convection and Diffusion application mode, using the Multiphysics feature in the Chemical Engineering Module.The Reynolds number is a dimensionless number obtained when formulating the Navier-Stokes equations in a dimensionless form. Regardless of the fluid properties and system geometry, the Reynolds number gives a measure of the convective momentum transport compared to the viscous momentum transport. The larger the convective term, the larger the Reynolds number. As the Reynolds number increases, the more turbulent the flow becomes, and the harder the problem is to solve numerically with the pure Navier-Stokes equations.The Reynolds number is defined as,where U is an average velocity of the flow in the system, ρ is the fluid’s density, and η is the dynamic viscosity. L is a characteristic length of the model geometry, usually a typical cross-sectional width for the flow. With a pipe, for example, L is its diameter. For complex geometries, however, a characteristic length might be difficult to determine.Flow is generally laminar for Re < 2300 and turbulent for Re > 4000. In the transition region between 2300 to 4000, the nature of the flow can be hard to determine and can be turbulent and/or laminar.You can estimate the Reynolds number in this model using water’s density, 1000 kg m -3, and its dynamic viscosity, 0.001 kg m -1 s -1. The inlet velocity is 0.1 m s -1.The difficulty in calculating an accurate Reynolds number typically lies in finding the length scale. However, you only need a rough estimate of the Reynolds number toRe ρUL η------------=8 | TU R B U L E N C E M O D E L I N G M I N I C O U R S Edetermine if the flow is turbulent or laminar (or in-between). In this geometry, a typical length could be the so-called “backstep” dimension, the height of one of the walls perpendicular to the inlet. L is here estimated to be 0.8 m. This results in Re = 80,000, which means that the flow is well within the turbulent flow regime.Although the flow is turbulent, you can begin by trying to apply the Incompressible Navier-Stokes equations to solve for the flow. You may consider this first step of the model to be a numerical experiment.M O D E L N A V I G A T O R1Start COMSOL Multiphysics.2In the Model Navigator set Space dimension to 2D .3Select Chemical Engineering Module>Momentum balance>IncompressibleNavier-Stokes .4Click OK .G E O M E T R Y M O D E L I N GThe figure below shows the reactor’s geometry.The 2D approximation implies that you neglect gradients in the directionperpendicular to the x -y plane (the z -direction). The 2D model is valid for a unit length thickness in the z -direction, in this case 1 m.The model geometry is inspired from the geometry of the reactor in a water-treatment process, where baffles are positioned between the inlet and outlet to create a tortuous path.Figure 2: The reactor geometry.1Hold the Shift key and click the Rectangle/Square button in the Drawtoolbar.outlet inlet2Enter rectangle properties according to the table and click OK:PROPERTY EXPRESSIONWidth5Height23Draw two more rectangles with these specifications:PROPERTY R2R3Width0.60.1Height0.41x-position-0.60.95y-position0.814Click the Zoom Extents button on the Main toolbar.5Select rectangle R2 and click the Array button on the Draw toolbar.6Set the value in the Displacement x edit field to 5.6 and set the Array size x to 2. Click OK.7Select rectangle R3 and click the Array button on the Draw toolbar.8Enter the following array properties, then click OK.PROPERTY EXPRESSIONDisplacement x1Displacement y0Array size x4Array size y19Select the two rectangles R5 and R7. Click the Move button on the Draw toolbar, and in the displacement:y edit field enter -1 to move the two objects by that amount.10Select R1, R2, and R4, then click the Union button on the Draw toolbar.11Select all the geometry objects by pressing Ctrl+A.12Click the Difference button on the Draw toolbar.13Finally, click the Delete Interior Boundaries button on the Draw toolbar.T U R B U L E N C E M O D E L I N G M I N I C O U R S E|910 | T U R B U L E N C E M O D E L I N G M I N I C O U R S EP H Y S I C S S E T T I N G SSubdomain SettingsThe stationary equations for the momentum balances and continuity are the Navier-Stokes equations defined as follows:where u denotes the velocity field (m s -1) and p pressure (Pa).You can use the properties for water, which are available from the material library that is supplied with COMSOL Multiphysics.1Select Subdomain Settings form the Physics menu.2Select subdomain 1 in the Subdomain selection list.3Click the Load button and select Water, liquid, from the Library 2 materials.4Click OK to close the Material/Coefficients Library window.5Click OK .Boundary ConditionsHere you specify an inlet velocity in the x -direction, u 0, as being 0.1 m s -1, and set the velocity in the y -direction, v , to zero. At the outlet, set pressure to zero. At all other boundaries, use the no slip condition (zero velocity). This is the default condition and applies to all walls.1From the Physics menu, open the Boundary Settings dialog box.2Enter the Boundary Settings from the following table:3Click OK .Note that you have not yet specified the constant u_in , which you do next through the Options menu. The remaining boundaries (2–27) have the default condition of no slip .BOUNDARY BOUNDARY CONDITION VARIABLE VALUE1Inflow/Outflow velocity u 0v 0u_in 028Pressure p 0∇p –I η+∇u ∇u ()T+()[]⋅–ρu ∇⋅()u +F=∇u ⋅0=TU R B U L E N C E M O D E L I N G M I N I C O U R S E | 11ConstantsSpecify the constants for temperature, used in the density and dynamic-viscosity functions from the material library, and define the inlet velocity u_in .1From the Options menu open the Constants dialog box.2Enter the following constant names, expressions, and descriptions:3Click OK .C O M P U T I N G T H E S O L U T I O NYou are now ready to try and solve the model.1Click the Solve button in the Main toolbar.After a few seconds the solver will stop and give you the following error message:2Click the Close button.The solver has failed to find a solution. The reason, of course, is that the flow is turbulent and there is no stable stationary solution using the pure Navier-Stokes equations. Even a time-dependent solution would probably require an immensely dense mesh to resolve the eddies in the flow. Simulations using the pure Navier-Stokes equations for turbulent flow (without using an averaging assumption for the turbulent fluctuations) are usually called direct numerical simulations and are typically solved on multiprocessor computers or clusters of computers with a comparably large amount of RAM. To proceed and solve the flow model you need to use a turbulence EXPRESSION DESCRIPTIONT 293.15Temperature [K]u_in 0.1Inlet velocity [m/s]12 | T U R B U L E N C E M O D E L I N G M I N I C O U R S ETurbulence models usually apply different averaging techniques to treat the effect of the turbulent eddies. The eddies play a very important role in transferring momentum within the fluid. In order to account for the momentum transport without resolving the eddies, turbulence models usually add a so-called eddy viscosity to increase the viscous momentum transport. This means that a momentum transport that, in reality, is convective, is averaged and translated to momentum transport by viscous forces. The eddy viscosity is usually calculated from the average flow field.The addition of eddy viscosity to the molecular viscosity decreases the effectiveReynolds number and removes the time-dependent turbulent variations allowing for a stationary solution of the “averaged” Navier-Stokes equations.The Reynolds-averaged representation of turbulent flows divides the flow quantities into an averaged value and a fluctuating part,(6)where φ can represent any of the velocity components or pressure. This is illustrated in the following figure:Figure 3: Reynolds averaging of velocity fluctuations due to turbulence.The velocity field, u i , is decomposed into one time-averaged contribution, U i , and one fluctuating part, u’i .This approach gives the Reynolds averaged equations below (Reynolds Averaged Navier-Stokes, RANS):φφφ′+=TU R B U L E N C E M O D E L I N G M I N I C O U R S E | 13where U is the averaged velocity vector.A comparison with the original Navier-Stokes equations indicates that the onlydifference is the appearance of the last term on the left-hand side, . This term includes the fluctuations around a mean flow and is called the Reynolds stress tensor. It is necessary to estimate the terms in this tensor in order to compute the mean flow. In RANS models, the Reynolds stresses are related to the average shear in the flow, using the following closure equation:where νT (m 2 s -1) denotes the eddy viscosity (sometimes referred to as the turbulent viscosity).A very simple approach is to change the viscosity in the Incompressible Navier-Stoke application mode by adding a constant eddy viscosity to the molecular (dynamic) viscosity over the whole domain. You do this by parametrically sweeping from a large turbulent viscosity to a low value and establish the lowest level that yield a converged solution.For this task you will use the parametric nonlinear solver in COMSOL Multiphysics. This solver starts with a parameter value, solves the problem, and uses this result as the initial guess for the next parameter value. In addition, it uses step-length control to swiftly sweep through the parameter list, so that it can take shorter steps as theproblems gets harder to solve. This procedure allows for a relatively fast convergence for highly nonlinear problems in general and here specifically for a convective problem. Below is the description of the usage in COMSOL Multiphysics.1From the Solve menu open the Solver Parameters dialog box.2From Solver list select Parametric .3On the General page, find the Name of parameter edit field and enter eta_T .ρt ∂∂Uη∇∇U ⋅–ρU ∇⋅U ∇P ρu 'u '⊗()∇+++F=∇U ⋅0=ρu 'u '⊗()∇u 'u '⊗νT –∇U ∇U ()T+()=4In the List of parameter values edit field enter logspace(1,-3,40).The logspace function generates a list of values, in this case spanning 101 to 10-3with 40 logarithmically equally spaced steps. This selection is very useful for thismodel.5Click OK to close the Solver Parameters dialog box.6Select the menu item Physics>Subdomain Settings.7In the Subdomain selection list choose 1.You can now modify the material properties, entering them manually instead ofusing the ready-made properties from the material library.8In the Density edit field enter 1000 (the density of water).9In the Viscosity edit field enter 1e-3+eta_T. Note that 1e-3 is the physical viscosityof water, and eta_T is the turbulent viscosity you add.10Click OKBefore solving the problem, you can also refine the mesh. This is in place to ensureaccurate results and to improve convergence.1Select the menu item Mesh>Free Mesh Parameters.2In the Global page select Extra Fine from the Predefined mesh sizes list.3Click Remesh and then OK. You should get a fine mesh, with approximately 2800elements. The number of elements is displayed in the Message Log.4Click the Solve button in the main toolbar to compute the parametric solutions.Thesolution process will take a minute or two.In the Progress - Solve Problem window you can see how the parametric solver worksthrough the list of parameter values. You might notice that the solver sometimesgoes back to a lower parameter value before proceeding just because the model 14|T U R B U L E N C E M O D E L I N G M I N I C O U R S ETU R B U L E N C E M O D E L I N G M I N I C O U R S E | 15becomes increasingly more difficult to solve as the parameter value (eddy viscosity) decreases.You find once again that the solver ends with an error report. This time it states that it could not converge for all parameter values.Remember that you are trying to push the problem to a level where the solution stops being stationary, where the solver should not find a unique solution. The procedure in this model gives a measure of the minimum turbulent viscosity for which the Navier-Stokes equations can be solved-for by COMSOL Multiphysics, dependent on the specified mesh density and the fact that eddy viscosity is constant in the domain.5Click Closeto continue with the model.P O S T P R O C E S S I N G A N D V I S U A L I Z A T I O NThe default plot shows the velocity field. Add streamlines to better visualize the flow.1Select the menu item Postprocessing>Plot Parameters. This opens the mainpostprocessing dialog box.2Go to the Streamline page. Select the Streamline plot check box, and in Number of start points edit field enter 50.3At the bottom of the Streamline page click the Line Color tab. Click the Color buttonand choose black. Click OK to close the Streamline Color dialog box.4Click OK to generate the following plotFigure 4: Final result for the velocity field from parametric solver.5Save this image for later use. Select the menu item File>Export>Image. Click Export,and in the File Name edit field supply a designation, for exampleResidence_result1. Then click Export.6Click Close.The final solved-for parameter value is the eddy viscosity for the final convergedsolution, i.e. the minimum value. You can find this value in the error message from thesolver, in the Log dialog box, or as a part of the title in the displayed plot.16|T U R B U L E N C E M O D E L I N G M I N I C O U R S E。
多层球形二次电子测量装置的栅网电子透过率仿真研究
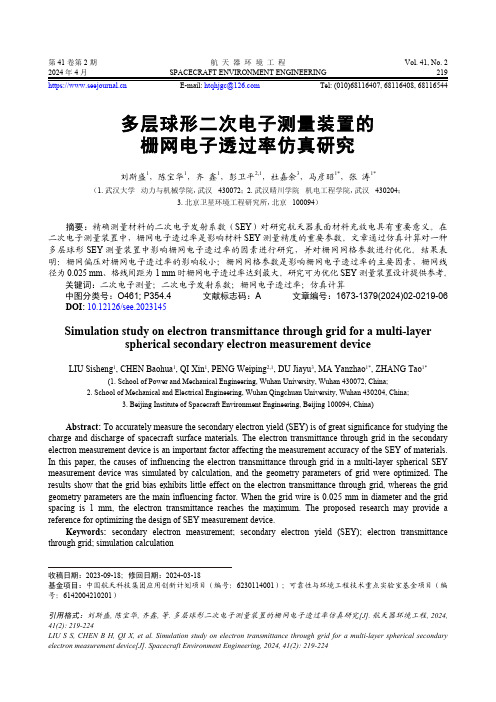
第 41 卷第 2 期航 天 器 环 境 工 程Vol. 41, No. 2 2024 年 4 月SPACECRAFT ENVIRONMENT ENGINEERING219 https:// E-mail: ***************Tel: (010)68116407, 68116408, 68116544多层球形二次电子测量装置的栅网电子透过率仿真研究刘斯盛1,陈宝华1,齐 鑫1,彭卫平2,1,杜嘉余3,马彦昭1*,张 涛1*(1. 武汉大学 动力与机械学院,武汉 430072; 2. 武汉晴川学院 机电工程学院,武汉 430204;3. 北京卫星环境工程研究所,北京 100094)摘要:精确测量材料的二次电子发射系数(SEY)对研究航天器表面材料充放电具有重要意义。
在二次电子测量装置中,栅网电子透过率是影响材料SEY测量精度的重要参数。
文章通过仿真计算对一种多层球形SEY测量装置中影响栅网电子透过率的因素进行研究,并对栅网网格参数进行优化。
结果表明:栅网偏压对栅网电子透过率的影响较小;栅网网格参数是影响栅网电子透过率的主要因素,栅网线径为0.025 mm、格线间距为1 mm时栅网电子透过率达到最大。
研究可为优化SEY测量装置设计提供参考。
关键词:二次电子测量;二次电子发射系数;栅网电子透过率;仿真计算中图分类号:O461; P354.4文献标志码:A文章编号:1673-1379(2024)02-0219-06 DOI: 10.12126/see.2023145Simulation study on electron transmittance through grid for a multi-layer spherical secondary electron measurement deviceLIU Sisheng1, CHEN Baohua1, QI Xin1, PENG Weiping2,1, DU Jiayu3, MA Yanzhao1*, ZHANG Tao1*(1. School of Power and Mechanical Engineering, Wuhan University, Wuhan 430072, China;2. School of Mechanical and Electrical Engineering, Wuhan Qingchuan University, Wuhan 430204, China;3. Beijing Institute of Spacecraft Environment Engineering, Beijing 100094, China)Abstract: To accurately measure the secondary electron yield (SEY) is of great significance for studying the charge and discharge of spacecraft surface materials. The electron transmittance through grid in the secondary electron measurement device is an important factor affecting the measurement accuracy of the SEY of materials. In this paper, the causes of influencing the electron transmittance through grid in a multi-layer spherical SEY measurement device was simulated by calculation, and the geometry parameters of grid were optimized. The results show that the grid bias exhibits little effect on the electron transmittance through grid, whereas the grid geometry parameters are the main influencing factor. When the grid wire is 0.025 mm in diameter and the grid spacing is 1 mm, the electron transmittance reaches the maximum. The proposed research may provide a reference for optimizing the design of SEY measurement device.Keywords: secondary electron measurement; secondary electron yield (SEY); electron transmittance through grid; simulation calculation收稿日期:2023-09-18;修回日期:2024-03-18基金项目:中国航天科技集团应用创新计划项目(编号:6230114001);可靠性与环境工程技术重点实验室基金项目(编号:6142004210201)引用格式:刘斯盛, 陈宝华, 齐鑫, 等. 多层球形二次电子测量装置的栅网电子透过率仿真研究[J]. 航天器环境工程, 2024, 41(2): 219-224LIU S S, CHEN B H, QI X, et al. Simulation study on electron transmittance through grid for a multi-layer spherical secondary electron measurement device[J]. Spacecraft Environment Engineering, 2024, 41(2): 219-2240 引言航天器表面材料充放电可能导致设备内部电子系统中的控制、指令以及数据等分系统发生异常或故障,严重时会危及航天器的正常运行[1-4]。
连接器专业英语
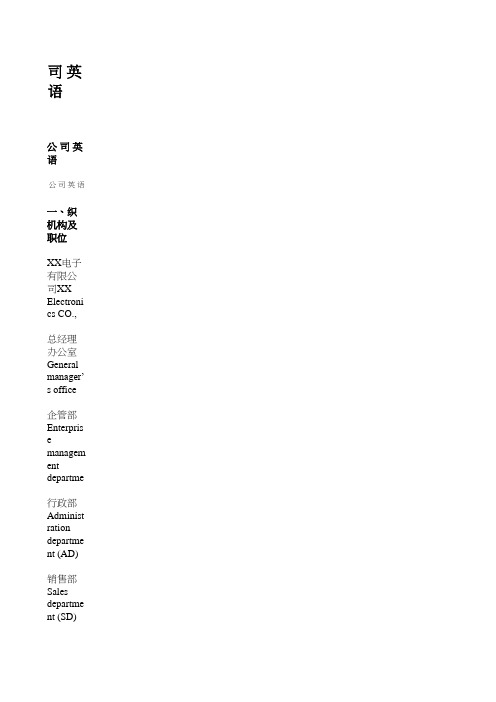
司 英 语公 司 英 语公司英语一、织机构及职位XX电子有限公司XX Electronics CO., Ltd总经理办公室General manager’s office企管部Enterprise management department (EM)行政部Administration department (AD)销售部Sales department (SD)财务部Financial department (FD)技术部Technology department (TD)物控部Production material control department (PMC)生产部Production department(PD)模具部Mold manufacturing department, Tooling manufacturing department (TM)品管部Quality Assurance department (QA)冲压车间Stamp workshop, press workshop注塑车间injection workshop装配车间Assembly workshop模具装配车间Mold and die Assembly workshop金属加工车间Metal machine workshop电脉冲车间Electric discharge process workshop线切割车间wire cutting process workshop 工磨车间Grinding workshop总经理General manager (GM)副总经理Vice-general manager经理manager董事长president副董事长Vice-presidentXX部门经理Manager of XX department 主任、主管supervisor拉长Line leader组长Foreman, forelady秘书secretary文员clerk操作员operator助理assistant职员staff二、产品连接器connector端子terminal条型连接器bar connector阴连接器Housing阳连接器wafer线束wire harness间距space额定电压 rated voltage额定电流 rated current接触电阻contact resistance绝缘电阻insulation resistance 超声波焊接ultrasonic welding 耐压withstand voltage针pin物料编号part number导线wire基体金属Base metal电缆夹cable clamp倒角chamfer接触面积contact area接触件安装孔contact cavity 接触长度contact length接触件电镀层contact plating 接触压力contact pressure接触件中心距contact space 接触簧片contact spring插孔socket contact法兰、击缘Flange界面间隙interfacial gap键Key键槽keyway过渡段ramp屏蔽套shielding定位基准Datum reference扁平电缆flat cable ,Ribbon cable柔性印刷电线Flexible printed wiring多层印制电路Multilayer printed circuit焊盘pad图形pattern间距pitch负极Negative pole正极positive pole回流Reflow原理图Schematic diagram单面板single sided board双面板Two-sided board,Double-sided board 表面安装surface Mounting翘曲warp, bow波峰焊wave soldering编织层braid同轴电缆coaxial cable电介质dielectric电缆中导线的头数ends外部干扰external interference填充物filler护套jacket比重specific gravity电阻的温度系数Temperature coefficient of resistance 三、模具塑料模具mould of plastics注塑模具injection mould冲压模具die模架mould base定模座板Top clamping plateTop plateFixed clamp plate水口推板stripper plateA板A plateB板B plate支承板 support plate方铁 spacer plate底针板 ejector plate面针板 ejector retainer plate回针 Return pin导柱 Guide pin有托导套 Shoulder Guide bush 直导套 Straight Guide bush动模座板Bottom clamp plate Moving clamp plate基准线datum line基准面datum plan型芯固定板core-retainer plate 击模固定板punch-retainer plate 顶针ejector pin单腔模具single cavity mould 多腔模具multi-cavity mould多浇口multi-gating浇口gate缺料starving排气breathing光泽gloss合模力mould clamping force锁模力mould locking force挤出extrusion开裂crack循环时间cycle time老化aging螺杆screw麻点pit嵌件insert活动镶件movable insert起垩chalking浇注系统feed system主流道 sprue分流道runner浇口gate直浇口direct gate , sprue gate轮辐浇口spoke gate , spider gate点浇口pin-point gate测浇口edge gate潜伏浇口submarine gate , tunnel gate 料穴cold-slug well浇口套sprue bush流道板runner plate排飞槽vent分型线(面)parting line定模stationary mould,Fixed mould 动模movable mould, movable half 上模upper mould, upper half下模lower mould, lower half型腔cavity凹模cavity plate,cavity block拼块split定位销dowel定位销孔dowel hole型芯core斜销angle pin, finger cam滑块slide滑块导板slide guide strip楔紧块heel block, wedge lock拉料杆sprue puller定位环locating ring冷却通cooling channel脱模斜度draft滑动型芯slide core螺纹型芯threaded core热流道模具hot-runner mould绝热流道模insulated runner mould熔合纹weld line (flow line)三板式模具three plate mould脱模ejection换模腔模具 interchangeable cavity mould 脱模剂release agent注射能力shot capacity注射速率injection rate注射压力injection pressure差色剂colorant保压时间holdup time闭模时间closing time定型装置sizing system阴模female mould,cavity block阳模male mould电加工设备Electron Discharge Machining 数控加工中心CNC machine center万能铁床Universal milling machine卧式刨床Horizontal planer车床Engine lathe平面磨床Surface grinding machine去磁机Demagnetization machine万能摇臂钻床Universal radial movable driller 立式钻床Vertical driller超声波清洗机Ultrasonic clearing machine 四、品管SPC statistic process control品管保证Quality Assurance品管控制Quality control来料检验IQC Incoming quality control巡检IPQC In-process quality control校对calibration动态试验dynamic test环境试验Environmental test非破坏性试验non-destructive test光泽gloss击穿电压(dielectric) breakdown voltage拉伸强度tensile strength冷热骤变试验thermal shock test环境试验炉Environmental chamber盐雾实验salt spray test绝缘电阻测试验仪Insulating resistance meter 内应力internal stress疲劳fatigue蠕变creep试样specimen撕裂强度tear strength缩痕shrink mark, sink mark 耐久性durability抽样sampling样品数量sample sizeAQL Acceptable Quality level 批量lot size抽样计划sampling planOC曲线operation curve试验顺序sequence of tests环境温度ambient temperature 可焊性solderability阻燃性flame resistance五、生产注塑机injection machine冲床Punch machine混料机blender mixer尼龙nylon黄铜 brass青铜 bronze紫(纯)铜 copper料斗hopper麻点pit配料compounding涂层coating飞边flash预热preheating再生料reworked material 再生塑料reworked plastics 工艺设计process design 紧急停止emergency stop 延时time delay。
双切环流气体分布器内流场FLUENT数值模拟_丰存礼

收稿日期:2003-10-29作者简介:丰存礼(1978-),男,山西朔州人,硕士研究生,主要从事化工设备和多相流体的研究工作。
文章编号:1000-7466(2004)02-0025-03双切环流气体分布器内流场FLUENT 数值模拟丰存礼,刘 成,李永辉,张敏华(天津大学石油化工技术开发中心,天津 300072)摘要:在FLUE NT 软件平台上,采用k -ε湍流模型模拟某实际应用的双切环流气体分布器内流体的流动状态。
对计算结果的分析以及与实际性能的对比表明,FLUE NT 可为双切环流气体分布器及其同类设备的新品研发、工业放大或优化改造提供方便快捷、功能强大的数值计算和理论分析。
关 键 词:气体分布器;数值模拟;计算流体力学;流场中图分类号:TQ 053 文献标识码:ANumerical simulation of the flow field in a twin -tangential annular flow gas distributor with F LUENTFE NG Cun -li ,LIU Cheng ,LI Yong -hui ,ZHANG Min -hua(Tianjin University R &D Center for Peterochem .Tech .,Tianjing Un iversity ,Tianjin 300072,China )A bstract :Three -dimensional flow field of a working twin -tangential annular flow gas distributor is simulated based on standardk -εturbulent model with FLUENT .The close agreement with the working data of the model predictions shows that FLUENT can provide a numerical description of fluid flow in the twin -tangential annular flow gas distributor within a considerable degree of accu -racy ,and FLUENT can also provide a convenient and powerful numeric calculation tool for exploitation ,research ,scale -up optimi -zation of gas distributor and congeners .Key words :gas distributor ;numerical simulation ;computational fluid dynamics ;flow field 塔器是炼油、化工等领域中广泛应用的设备,在塔器底部常设有促使气体均匀分布的进气装置———气体分布器。
Flow visualization.docx
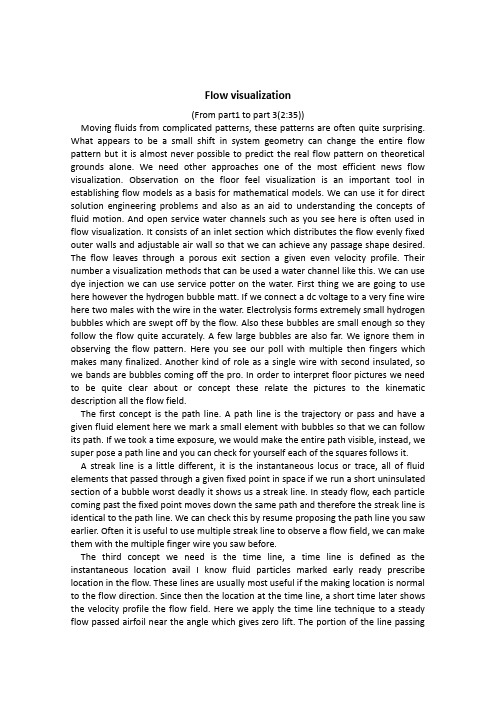
Flow visualization(From part1 to part 3(2:35))Moving fluids from complicated patterns, these patterns are often quite surprising. What appears to be a small shift in system geometry can change the entire flow pattern but it is almost never possible to predict the real flow pattern on theoretical grounds alone. We need other approaches one of the most efficient news flow visualization. Observation on the floor feel visualization is an important tool in establishing flow models as a basis for mathematical models. We can use it for direct solution engineering problems and also as an aid to understanding the concepts of fluid motion. And open service water channels such as you see here is often used in flow visualization. It consists of an inlet section which distributes the flow evenly fixed outer walls and adjustable air wall so that we can achieve any passage shape desired. The flow leaves through a porous exit section a given even velocity profile. Their number a visualization methods that can be used a water channel like this. We can use dye injection we can use service potter on the water. First thing we are going to use here however the hydrogen bubble matt. If we connect a dc voltage to a very fine wire here two males with the wire in the water. Electrolysis forms extremely small hydrogen bubbles which are swept off by the flow. Also these bubbles are small enough so they follow the flow quite accurately. A few large bubbles are also far. We ignore them in observing the flow pattern. Here you see our poll with multiple then fingers which makes many finalized. Another kind of role as a single wire with second insulated, so we bands are bubbles coming off the pro. In order to interpret floor pictures we need to be quite clear about or concept these relate the pictures to the kinematic description all the flow field.The first concept is the path line. A path line is the trajectory or pass and have a given fluid element here we mark a small element with bubbles so that we can follow its path. If we took a time exposure, we would make the entire path visible, instead, we super pose a path line and you can check for yourself each of the squares follows it.A streak line is a little different, it is the instantaneous locus or trace, all of fluid elements that passed through a given fixed point in space if we run a short uninsulated section of a bubble worst deadly it shows us a streak line. In steady flow, each particle coming past the fixed point moves down the same path and therefore the streak line is identical to the path line. We can check this by resume proposing the path line you saw earlier. Often it is useful to use multiple streak line to observe a flow field, we can make them with the multiple finger wire you saw before.The third concept we need is the time line, a time line is defined as the instantaneous location avail I know fluid particles marked early ready prescribe location in the flow. These lines are usually most useful if the making location is normal to the flow direction. Since then the location at the time line, a short time later shows the velocity profile the flow field. Here we apply the time line technique to a steady flow passed airfoil near the angle which gives zero lift. The portion of the line passingabove your for oil rejoins the portion passing beneath at the trailing edge except for the narrow boundary their region where the flow is retarded by friction. Since the distance around either side of the airfoil is approximately the same. The time line show that the average speed above the airfoil is approximately the same as beneath it. When the airfoil is set at angles which give large lift, a portion of the time line passing above the airfoil out runs the portion bunny the velocity is higher near the upper surface. At negative incidents, the effects are reversed.The fourth concept we need is the streamline. A streamlined Estefan as any line at a given instant is everywhere attention to the velocity vectors are the flow field. The streamline is essentially a mathematical idea and is very useful in many polo analyses, but there’s n o way to take a streamlined directly visible. You see in steady flow, the pipeline industry Klein are the same and you might suspect. But the streamlines would coincide with them also this is in fact the case. Consider a particle moving along unsteady flow as you saw each particle moves down the same path line so that always moving down this path towards the particle headed, this means it is always change into the path and this is how we define to streamline. So in steady flow, the streamline, the speak line, in the path line are all the same. And we can use either streak lines or path lines to show us the streamlined butter. Another thing we often need is the velocity vector field itself. We can find the velocity feel by using what I call combine pine street markers. The bubble squares you see here are such markers may have a number a useful properties and you can prove for yourself. The side to the markers are streak lines. In study flow all the sides show the stream on pattern. The front and back of the markers our timelines in a two dimensional incompressible flow area of each maker is forever constant. Since the time interval for a given maker to pass any fixed point in space is also constant. The link from the front to the back of a marker at any location is a major average velocity in that region.Now consider for a moment and unsteady flow, like the one you see here passed an awesome letting play.Do you think in this case the streak alive or the path lined will be the same as the streamlines? Let’s look it up path line and see. Here’s a single mark mellman entering a fixed time in a cycle of play. As before, we superposed its path line. I will compare a path line with a succession of instantaneous streak lines entering through the same point. None the instantaneous streak lines Cohen saws with the path line. Here is a second loan entering through the same point but starting at a different time in the cycle of the plate told us that if path line crosses that on the first element almost at right angles and it leaves around the other side of the plate. Now you see elements mark at both times in the cycle on the play. If we superposed the path lined up the second element and compare it with a set of strict line through the same point, you can see that love them coincide with the second path lined either.Now let’s see about streamlines. In order to fine the streamlines, we need the direction of the velocity at each point; we can find this from combined time street markers such as you see here. If we take a transparency, a one frame a multiple timesstreak lines and overlay on it another transparency taken a few moments later. We can connect the end points above the streak lines and shoulder particle pass for short time intervals. Here you see the short dashes made by connecting the end points at the time streak lines; they show us the velocity direction at each point. If we draw lines parallel to them, these are the streamlines. The tall streamline picture you see here is for the same instant but the first particle we observed with the path line entered the frame. A daughter and streamline is the one that passes through the same point. If we compare the dotted streamline with the path line through the same point and at the same instant in time, you see they’re quite different. If we compare the dotted streamline when the set us free Klein’s entering through the same point, they do not coincide either.In a transient flow the streak line, the pipeline and the streamline are all different if the streak lines move side wise as you see here, then the flow is transient and we cannot use the streak lines for streamline. In a transient flow we must work out the velocity field from some kind of combined time streak markers. We have seen one way to make the flow visible, policy how to use this information to solve engineering problems and as a basis for mathematical analysis. As an example, let’s consider straight wall diffusers as you probably know it if users any passage to decelerate the flow and causes a rise in pressure. In subsonic flow, the diffuser is merely a diverging passage. Between 1990 and about 1950, a number study showed that if we increase the divergence angle the diffuser 2θ, but the pressure rises increased up to some modest angle, but further increases and angle cause the pressurized to decrease. At that time we still did not have however any good picture the overall neither flow patterns, nor did we have a good methods for predicting of non-performance. We then began a series of systematic visual studies and these quickly lead to better understanding and to improve predictive methods. The bubble technique can show us what these patterns actually are.Let’s start with the walls parallel, notice how the velocity drops sharply near the wall and in this region the particle slide evenly over each other. This low velocity region is called a laminar boundary layer, knowledge open up the diffuser to a total angle about seven degrees. The increasing area of the passage causes the velocity to decrease and their pressure to rise in the direction of flow. Watch a streak square in the middle of the passage, you can see the deceleration. Also noticed the floor near the wall, you see a thicker boundary there now and it is no longer steady instead you see here regular time-dependent Eddie’s. This kind of boundary layer is called turbulent even through some other particles very near the wall do not have enough momentum to overcome the rising pressure. They mix with the particles father from the wall and are given sufficient momentum to keep moving in the downstream direction.If we increase the divergence angle diffuser further, something new occurs slow moving particles begin to accumulate near one wall and then move upstream forming what we call a stall. The mainstream is forced to separate from the wall and move around the stall, the pressure change caused by the movement in the mainstream isenough so that after a short time the stall washes out again. The whole cycle has stalled buildup in wash out, then repeats in time. These unsteady motions we call large transit or stall, in this regime the whole passage acts like an awesome later, even though the upstream flow is steady and summer trick.If we increase the divergence angle the diffuser still further, the stall forms a relatively steady recirculating region on one wall. The mainstream separates very near the flow and hugs the other wall. If we move the mainstream to the opposite wall it stays there. The pattern is what we call by a stable usually we refer to this pattern as fully develops Paul. If we increase the diffuser angle too high values, the flow separates from both walls. We observe a jet flow pattern.In studying fluid patterns, it’s important to examine the whole flow filed. Here’s a diffuser with the lower divergence angle, bubble water near the surface overflow shows an entirely on stall low power.Now we will turn off this wire near the surface and turn on another one deep in the flow near the floor the channel. This wire shoulders than one corner the flow contains a large tall area. The wire near the surface still shows on stalled flow. If we use this wire alone it would seriously have misled us about the nature the flow pattern like any other experimental tool. Flow visualization must be used with care to obtain reliable results. Knowledge about flow patterns is most useful. When summarized in the form of a correlation in the case of our diffuser problem, we can show the summary new by this chart. It shows divergence angle to fade a plotted versus length to width ratio like the user divided by the W, the chart shows us, when we will find each other for flow regimes you seen in terms of the parameters. These lines indicate ships from one flow regime to another. One point that needs emphasis about the ship’s implosion is that it’s very solemn that we can predict them on theoretical grounds alone, even when we have a good flow model and a sound theory based on it. Within a given regime we may not be able to predict where that regime ends. This is the case in the lowest region. In this region if we stay well below the liner first all, then we can use primal potential theory boundaries model to make accurate predictions about the flow in about performance, but there is presently available nor theory that will protect the transition. That is this whole line higher up on the chart in the other regions. The theories are not as complete and there is no theory that will predict. This line for the history says region either.We might summarize all is as follows: when we first began to study a problem in fluid mechanics, we may not even know the gross flow patterns to expect. After he became mad enough systematic information in visual former by other means, we can correlate the data as for example on the chart. Finally, we can use the flow model so file as the basis for mathematical models and if these are successful, we can now make paper and pencil predictions about a good many details. We can do that in this regime on the diffuser char. the information inherent in the second record elation stage is often useful for other purposes. Sometimes it tell us how to locate of the moments and sometimes it suggests improved disarm. In the diffuser example, lost line in fact tells usthe geometry is that give maximum pressure rise for a fixed like the diffuser; the chart itself also suggests a way to improve short diffusers. Short diffuser usually mean wide angles but wide angles mean separations that are patterns and poor performance. The charts suggest that perhaps we ought what we ought to do is to divide up the passages into smaller passages each one of which does not cross the line first line up stall either this way for this way.Let’s see if this idea works. Here you see a fully develop stall in a diffuser with the divergence angle about 45 degrees, but in certain assembly or veins designed so that each passage lies below the liner first appreciable separation on our floor regime chart. When the veins are removed, the stall comes back; it is often to use more than one method visualization. Let’s use another technique to look at some other things we have seen before and also to study a new pattern of Lauren jeans laminar turbulent transition.。
压电薄膜加速度计-eTouch
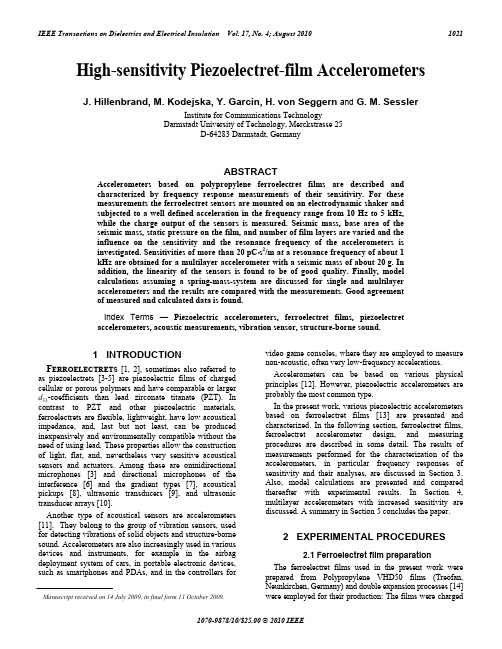
High-sensitivity Piezoelectret-film AccelerometersJ. Hillenbrand, M. Kodejska, Y. Garcin, H. von Seggern and G. M. SesslerInstitute for Communications TechnologyDarmstadt University of Technology, Merckstrasse 25D-64283 Darmstadt, GermanyABSTRACTAccelerometers based on polypropylene ferroelectret films are described andcharacterized by frequency response measurements of their sensitivity. For thesemeasurements the ferroelectret sensors are mounted on an electrodynamic shaker andsubjected to a well defined acceleration in the frequency range from 10 Hz to 5 kHz,while the charge output of the sensors is measured. Seismic mass, base area of theseismic mass, static pressure on the film, and number of film layers are varied and theinfluence on the sensitivity and the resonance frequency of the accelerometers isinvestigated. Sensitivities of more than 20 pC⋅s2/m at a resonance frequency of about 1kHz are obtained for a multilayer accelerometer with a seismic mass of about 20 g. Inaddition, the linearity of the sensors is found to be of good quality. Finally, modelcalculations assuming a spring-mass-system are discussed for single and multilayeraccelerometers and the results are compared with the measurements. Good agreementof measured and calculated data is found.Index Terms — Piezoelectric accelerometers, ferroelectret films, piezoelectretaccelerometers, acoustic measurements, vibration sensor, structure-borne sound.1 INTRODUCTIONF ERROELECTRETS [1, 2], sometimes also referred to as piezoelectrets [3-5] are piezoelectric films of charged cellular or porous polymers and have comparable or larger d33-coefficients than lead zirconate titanate (PZT). In contrast to PZT and other piezoelectric materials, ferroelectrets are flexible, lightweight, have low acoustical impedance, and, last but not least, can be produced inexpensively and environmentally compatible without the need of using lead. These properties allow the construction of light, flat, and, nevertheless very sensitive acoustical sensors and actuators. Among these are omnidirectional microphones [3] and directional microphones of the interference [6] and the gradient types [7], acoustical pickups [8], ultrasonic transducers [9], and ultrasonic transducer arrays [10].Another type of acoustical sensors are accelerometers [11]. They belong to the group of vibration sensors, used for detecting vibrations of solid objects and structure-borne sound. Accelerometers are also increasingly used in various devices and instruments, for example in the airbag deployment system of cars, in portable electronic devices, such as smartphones and PDAs, and in the controllers for video game consoles, where they are employed to measure non-acoustic, often very low-frequency accelerations. Accelerometers can be based on various physical principles [12]. However, piezoelectric accelerometers are probably the most common type.In the present work, various piezoelectric accelerometers based on ferroelectret films [13] are presented and characterized. In the following section, ferroelectret films, ferroelectret accelerometer design, and measuring procedures are described in some detail. The results of measurements performed for the characterization of the accelerometers, in particular frequency responses of sensitivity and their analyses, are discussed in Section 3. Also, model calculations are presented and compared thereafter with experimental results. In Section 4, multilayer accelerometers with increased sensitivity are discussed. A summary in Section 5 concludes the paper.2 EXPERIMENTAL PROCEDURES2.1 Ferroelectret film preparationThe ferroelectret films used in the present work were prepared from Polypropylene VHD50 films (Treofan, Neunkirchen, Germany) and double expansion processes [14] were employed for their production: The films were chargedManuscript received on 14 July 2009, in final form 11 October 2009.1070-9878/10/$25.00 © 2010 IEEEafter the first thickness expansion for 60 s with a point-to-plate corona setup and a needle voltage of 32 kV. Thereafter, the samples were metalized on both sides and the second expansion was performed. High sensitivities and high resonance frequencies of the accelerometers are obtained when ferroelectrets with large d 33-coefficients and large Young’s moduli are used. Since the optimal expansion parameter set (T 1, T 2, p 1, and p 2: temperature and pressure of first and second expansion, respectively) for this combination of large coefficient and modulus is unknown, most of the films used in this study were prepared with the parameter set T 1=90 °C, p 1=2 MPa (20 bar), T 2=60 °C and p 2= 2 MPa (20 bar) [15] which is known to result in relatively soft films with high d 33-coefficients. However, films prepared with other parameter sets, leading to ferroelectrets with lower d 33-coefficients but higher Young’s moduli, were also employed in the present work.2.2 AccelerometersFor the basic studies on ferroelectret accelerometers, presented in the following, mainly two different designs were used. Both designs are relatively large and heavy and are not hermetically sealed. Schematic pictures of the two designs are shown in Figure 1a and 1b. In the design of Fig. 1a, a spring/screw-combination exerts a static force to the center of the seismic mass and in the design of Figure 1b, two spring/screw-combinations act on the two sides of a metal arbor which in turn is in direct contact to the seismic mass.seismic mass ferroelectret-film baseplateseismic mass ferroelectret-filmbaseplatehousing spring/screw (force adjustable)seismic mass ferroelectret-film baseplatehousing spring/screws (forces adjustable)Figure 1. Three designs of ferroelectret accelerometers: In the first design (1a), a single spring/screw-combination generates the static force while in the second design (1b), two spring/screw-combinations act via a metal arbor on the seismic mass. In the most simple design (1c) the seismic mass is glued on the ferroelectret which in turn is glued on the vibrating object. In all three designs quadratic seismic masses with an edge length of 1.7 cm and various heights were used.In ferroelectret accelerometers as in many other piezoelectric accelerometers, the basic task of the static force is to anchor the seismic mass in such a way thatvibration of the mass in measuring direction is not hindered but any movement of the mass in other directions is strongly restricted. A static force to the seismic mass generates an equal static force to the film. Such a force changes the d 33-coefficient and Young’s modulus of the film and therefore sensitivity and resonance frequency of a ferroelectret accelerometer. Measurements demonstrating these effects are shown in the following.A big advantage of the designs in Figures 1a and 1b is their flexibility: Ferroelectret films and seismic masses can be easily changed and the number of film layers of a folded film, the size and base area of the seismic mass and the value of the static force to the film can be adjusted in a wide range.With ferroelectret films it is even possible to construct very simple accelerometers without any housing by gluing the seismic mass directly to the film (Figure 1c) and omitting any static pressure to the mass. However, in the present study static pressures were always applied to the films during all measurements.Smaller and lighter accelerometers, with housings hermetically sealed and thus protecting the ferroelectret films from acoustic and electrical noise, are presently under investigation in another study. Their construction strongly depends on the conclusions drawn from the accelerometer designs used in the present work. Results obtained from these smaller accelerometers with soundproofed housings will be presented elsewhere.2.3 Acceleration measurementsFor the measurements, the accelerometers described above were mounted on an electrodynamic vibration exciter (B&K 4809). The exciter is driven by a power amplifier (B&K 2713). The charge generated by the accelerometer is amplified by a charge amplifier (B&K 2635). An audio analyzer (R&S UPD) was used to generate the signal for the power amplifier and to record and analyze the output signal of the charge amplifier.Typically, sinusoidal excitation signals were used in the range from 10 Hz to 5 kHz and the output signal of the charge amplifier was filtered with a digital bandpass before the amplitude was calculated. In some experiments, white noise was used as an excitation signal and the output of the charge amplifier was analyzed with a Fast Fourier Transform (FFT). As expected, both methods yield very similar frequency responses for the tested accelerometers.The entire measuring setup described above does not have an ideal flat frequency response. This is essentially due to the electrodynamic principle of the vibration exciter. For a constant input voltage, the resulting acceleration significantly decreases below 100 Hz and above a resonance of the shaker at about 10 kHz.Therefore, calibration measurements of the setup were performed using commercial accelerometers (B&K 4332 and 4344). The smaller weights of the commercial accelerometers, compared to the ferroelectretaccelerometers, were compensated by mounting additional masses on the shaker during the calibration measurements, which ensures that in this case the same acceleration is generated by the shaker.Usually, the frequency dependent acceleration generated by the shaker was accepted and finally compensated numerically by the calibration curves. However, if linearity was tested or non-linear effects were expected or observed, in particular non-linear behavior of Young’s modulus of the ferroelectret films, the equalization possibility of the audio analyzer was utilized: Measuring the frequency response of the output signal in a first step and applying the inverse response to the input in further measurements assures relatively frequency independent accelerations at the shaker.3 SENSITIVITY MEASUREMENTS3.1 Dependence on seismic massSensitivity measurements of an accelerometer of the two-spring design are shown in Figure 2. The various measurements were performed with different masses from 3.7 to 21.8 g. The base area for all masses was 2.9 cm 2 and the static force generated by the two springs was also kept constant at a value of 12 kPa.For each measurement, a slight decrease of the sensitivity with increasing frequency followed by a resonance peak was found. The decrease of sensitivity is due to the typical decrease of the d 33-coefficient of the ferroelectret films [16, 17]. The resonance peak can be understood when the film, with Young’s modulus Y , and the seismic mass m s is considered as a spring-mass-system.S e n s i t i v i t y [p C /m /s 2]Frequency [Hz]Figure 2. Sensitivity of an accelerometer with different seismic masses. For all measurements the same ferroelectret film was used and the same static pressure was applied. The quasistatically measured d 33 is 600 pC/N.From Figure 2 it can also be seen that the sensitivity of an accelerometer increases and its resonance frequency decreases, when the seismic mass is enlarged. As mentioned before, modeling the accelerometers as spring-mass-systems can explain these findings. This will be shown in the following.From the definition of the charge sensitivity S of an accelerometer, the definition of the d 33-coefficient, andNewton’s second law it follows that S can be written ass 33m d aQS ⋅==(1) with generated charge Q and applied acceleration a . Equation (1) shows that the sensitivity of an accelerometer S is linearly dependent on the d 33-coefficient of the film and on its seismic mass m s .The resonance frequency πω2/res res =f of a spring-mass-system is given by m D /res =ω with the stiffness t YA D /=, where Young’s modulus is defined as )/()(t A Ft Y Δ⋅=, A being the base area of the seismic mass and t the thickness of the ferroelectret film. Therefore, the resonance frequency of the accelerometer can be written astm Ap Y f ⋅⋅=s s res )(21π(2)where Y (p s ) has been written for Young’s modulus since it depends on the applied static pressure p s , if p s > 20 kPa (see sect. 3.2).Calculated values from both equations are compared in Figure 3 with measured values obtained from the data presented in Figure 2. Measured sensitivities (at 100 Hz) and resonance frequencies are shown as symbols and the two solid lines are the results obtained from equations (1) and (2). Both, the linear and the inverse root dependencies are in good agreement with the measured data points.12345Resonance frequency [kHz]S e n s i t i v i t y [p C /m /s 2]Seismic mass [g]Figure 3. Sensitivity (@100 Hz) and resonance frequency from the various curves shown in Figure 2 (symbols) and from equations (1) and (2) (solid lines).The straight line for the sensitivity was obtained from equation (1) for a d 33-coefficient of 405 pC/N. This value at 100 Hz is in reasonable agreement with the 600 pC/N determined quasistatically on the ferroelectret before the film was mounted into the accelerometer. The difference between both values is due to the well known decrease of d 33 with increasing frequency [16, 17].The inverse root function for the resonance frequency was obtained from equation (2) for a film thickness of 60 µm, a seismic mass base area of 2.9 cm 2, and a Young’smodulus of 0.52 MPa. While the first two values are just measured data, the value for Young’s modulus was obtained as the fitting parameter from the plotted inverse root function. Its value is very reasonable for a double expanded PP film [18].3.2 Dependence on static pressureThe basic idea of applying a static pressure, which can be quite large compared to the acceleration forces, is to ensure a stable bilateral fastening of the seismic mass on the inside top and bottom of the accelerometer housing without hindering the seismic mass to move and to vibrate in the direction perpendicular to the film plane.The static pressure influences sensitivity and resonance frequency of the ferroelectret accelerometers. While the dependence of these parameters on the seismic mass is obvious and easy to explain by a spring-mass-system (see above), their dependence on the static pressure is more complicated. This will be shown in Figures 4 and 5.In Figure 4 various frequency response measurements of the sensitivity for static pressures from 0 to 50 kPa are shown. For all measurements a seismic mass of 10.2 g, an area of 2.9 cm 2, and a ferroelectret film with a d 33 of 600 pC/N (quasistatically determined) was used.S e n s i t i v i t y [p C /m /s 2]Frequency [Hz]Figure 4. Sensitivity of an accelerometer with a seismic mass of 10.2 g. The static pressure on the seismic mass was changed in the range from 0 to 50 kPa. For all measurements the same ferroelectret-film with a quasistatically determined d 33 of 600 pC/N was used.Again, as for the measurements with various seismic masses, a slight decrease of the sensitivity with increasing frequency followed by a relatively flat part and a resonance peak were found for each measured frequency response. It is interesting to note that the sensitivity at frequencies well below the resonance peak does not depend on the static pressure as long as it is below about 20 kPa.For the illustration of this more complicated behavior, the results of Figure 4 are summarized in Figure 5: The sensitivity at 200 Hz and the resonance frequency are presented in the Figure as a function of the applied static pressure.While the resonance frequencies directly increase with increasing static pressure, the sensitivities are initially constant up to a pressure of about 20 kPa and then start to decrease with increasing pressure. The behavior of sensitivity and resonance frequency as a function of the static pressurecan be explained if two effects, namely the quite rough surface of the ferroelectret films and their non-linear Young’s modulus are taken into account: With increasing static pressure the effective contact area of the films increases. This does not change the sensitivity S , since S is proportional to d 33 (see equation (1)) and d 33 does not depend on the applied pressure for small and medium pressures up to 10 kPa [16]. However, the resonance frequency does change, since restoring forces increase with increasing contact area and the resonance frequency therefore increases.Resonance frequency [kHz]S e n s i t i v i t y [p C /m /s 2]Static pressure [kPa]Figure 5. Sensitivity (at 200 Hz) and resonance frequency of the various frequency responses presented in Figure 4.The decrease of sensitivity and the more pronounced increase of the resonance frequency above 25 kPa is due to a non-linear behavior of Young’s modulus Y of the ferroelectret film. As Y increases with higher pressure, the resonance frequency increases [17] and the d 33-coefficient as well as the proportional sensitivity S decrease.3.3 Linearity of sensitivityIn this section two series of measurements are presented, which both demonstrate the excellent linearity of ferroelectret accelerometers.In the first series, frequency responses of the sensitivity were measured for an accelerometer in which the seismic mass and the static pressure were kept constant but the contact area between the seismic mass and the ferroelectret film were varied by using adaptors. The measurements are shown in Figure 6.For frequencies below 300 Hz, the frequency responses for all contact areas are very similar. This is not the case for higher frequencies, since the resonance frequencies increase with increasing contact area A . An examination of the resonance frequencies in Figure 6 shows that their increase with A is well described by a root function, as predicted by equation (2).In the second series of linearity measurements, shown in Figure 7, frequency responses of the sensitivity were determined for different accelerations ranging from 1.15 to 11 m/s 2. The equalization feature of the audio analyzer was utilized to obtain a frequency-independent acceleration at the shaker. All four frequency responses coincide, i.e. perfect linearity in the given acceleration range is assured. Note that the acceleration range given above is due to thelimits of the shaker. It is thus much smaller than the full linear range of a ferroelectret accelerometer. It is estimated that this range extends at least up to 300 m/s 2 for a seismic mass of 10 g.S e n s i t i v i t y [p C /m /s 2]Frequency [Hz]Figure 6. Sensitivity of an accelerometer with a seismic mass of 10.2 g. The contact area of the seismic mass was changed in the range from 0.72to 2.9 cm 2. For all measurements the same ferroelectret-film with a quasistatically determined d 33 of 700 pC/N was used.S e n s i t i v i t y [p C /m /s 2]Frequency [Hz]Figure 7. Sensitivity of an accelerometer with a seismic mass of 10.2 g and a ferroelectret-film with a quasistatically determined d 33 of 700 pC/N. Four different accelerations from 1.15 to 11 m/s 2 were applied to the accelerometer during each frequency response measurement of the sensitivity. Note that the shaker does not allow high accelerations at low frequencies. Therefore, the starting frequency is 100 Hz.4 MULTILAYER ACCELEROMETERSFolding ferroelectret-films once or multiple times yields zigzag-shaped multilayer (or stacked) transducers. When a seismic mass is mounted on such a folded stack and accelerated, inertial forces will act on each of the layers. Therefore, n times more charges are generated (n : number of layers) than for a single layer system. However, a stack of several films is n times thicker and thus “softer” than a single film and hence the resonance frequency of a multilayer accelerometer is smaller than that of a single layer sensor.With these findings, equations (1) and (2) can be rewritten for multilayer sensors:s 33m d n aQS ⋅⋅== (3) t n m Ap Y f ⋅⋅⋅=s s res )(21π (4) Sensitivity measurements of a two-layer accelerometer of the two-spring design are shown in Figure 8. Seismic masses from 3.7 to 25.5 g were used. Seismic mass area and static pressure were kept constant at values of 2.9 cm 2 and 12 kPa, respectively.The results are very similar as for single layer accelerometers. In particular, increasing the sensitivity byincreasing the seismic mass also results in a decreasing resonance frequency. However, with multilayer accelerometers, the same sensitivity/resonance frequency pairs can be obtained with smaller seismic masses, resulting in lighter accelerometers.110S e n s i t i v i t y [p C /m /s 2]Frequency [Hz]Figure 8. Sensitivity of a two-layer accelerometer with different seismic masses. For all measurements the same two layers of a ferroelectret-film (d 33=475 pC/N) were used and a static pressure of 12 kPa was applied.A comparison of measured sensitivities (at 100 Hz) and resonance frequencies obtained from Figure 8 (circles and triangles) and results obtained from equations (3) and (4) (the two lines) is shown in Figure 9.Resonance frequency [kHz]S e n s i t i v i t y [p C /m /s 2]Seismic mass [g]Figure 9. Sensitivity (at 100 Hz) and resonance frequency of the various curves shown in Figure 8. The lines are calculated by eqs. (3) and (4).Both theoretical functions are in good agreement with the measured values. A d 33-coefficient of 360 pC/N and a Young’s modulus of 0.48 MPa were obtained as fitting parameters from the two functions. Both values are very reasonable and in close accord with quasistatically determined values (475 pC/N before the film was mounted into the accelerometer) or with literature data [17], respectively.Investigations were also made with three-layeraccelerometers of the two-spring design and seismicmasses from 3.7 to 21.8 g were used. Seismic mass area and static pressure were kept constant. The measurements are shown in Figure 10, a comparison of experimental data with model results is presented in Figure 11.110100S e n s i t i v i t y [p C /m /s 2]Frequency [Hz]Figure 10. Sensitivity of a three-layer accelerometer with different seismic masses. For all measurements the same two layers of a ferroelectret-film (d 33=470 pC/N) were used and a static pressure of 12 kPa was applied.In general, the frequency responses of the sensitivity are comparable to results obtained from single- and two-layer accelerometers. However, as expected from equations (3) and (4), sensitivities are higher and resonance frequencies are lower. Note that high sensitivities of more than 20 pC ⋅s 2/m were obtained with seismic masses of only about 20 g.From Figure 11 a d 33-coefficient of 330 pC/N and a Young’s modulus of 0.48 MPa were obtained as fitting parameters. Again, these values are in reasonable agreement with the expected values.Resonance frequency [kHz]S e n s i t i v i t y [p C /m /s 2]Seismic mass [g]Figure 11. Sensitivity (at 100 Hz) and resonance frequency for the various curves shown in Figure 10. The lines are calculated by equations (3) and (4).Finally, three more frequency responses of accelerometers are presented in Figure 12. The accelerometers were designed with a single stripe of ferroelectret film, first folded two times and used as a three-layer sensor, then cutting off one layer and using it as a two-layer sensor and finally, after a second cut, as a single-layer accelerometer. This Figure impressively demonstrates the behavior and the advantages and disadvantages with respect to sensitivity and resonance frequency of multilayer sensors at a glance.S e n s i t i v i t y [p C /m /s 2]Frequency [Hz]Figure 12. Sensitivities of accelerometers with one, two, and three layers of ferroelectret-film (d 33=500 pC/N). In all three accelerometers a seismic mass of 10 g and a static pressure of 12 kPa were used.5 SUMMARYAccelerometers based on ferroelectrets are lightweight and inexpensive. Their sensitivity and resonance frequency can be adjusted by the d 33-coefficient and Young’s modulus of the film and by the seismic mass, the static pressure on the film, and the number of films. Presently, sensitivities of more than 20 pC ⋅s 2/m are available.Two further advantages of ferroelectret accelerometers can be stated: Since the conductivity of the ferroelectret films is very low - electret charges are stable for years - the output charges of ferroelectret films are dissipated extremely slowly and the devices can therefore be used at very low frequencies, much below the frequency range investigated in the present paper. Furthermore, temperature changes generate only few charges, since the pyroelectric coefficient of ferroelectrets is quite small [4], one to two orders of magnitude smaller than that of PZT. Therefore, the output signals of ferroelectret accelerometers are rather insensitive to temperature variations.Finally it should be mentioned that all measurements are in good agreement with model calculations. Ferroelectret accelerometers are thus theoretically well understood.ACKNOWLEDGEMENTSThe authors gratefully acknowledge financial support by the Deutsche Forschungsgemeinschaft and the Hessische Ministerium für Wissenschaft und Kunst.REFERENCES[1]S. Bauer, R. Gerhard-Multhaupt, and G. M. Sessler, “Ferroelectrets: Soft Electroactive Foams for Transducers“, Physics Today, Vol. 57, pp. 37-43, 2004.[2]M. Wegener, W. Wirges, R. Gerhard-Multhaupt, M. Dansachmüller, R. Schwödiauer, S. Bauer-Gogonea, S. Bauer, M. Paajanen, H. Minkkinen, and J. Raukola, “Controlled inflation of voids in cellular polymer ferroelectrets: Optimizing electromechanical transducer properties”, Appl. Phys. Lett. Vol. 84, pp. 392-394, 2004.[3]J. Hillenbrand and G. M. Sessler, “High-sensitivity piezoelectric microphones based on stacked cellular polymer films”, J. Acoust. Soc. Am., Vol. 116, pp. 3267-3270, 2004.[4]S. Bauer, “Piezo-, pyro- and ferroelectrets: soft transducer materials for electromechanical energy conversion”, Trans. Dielectr. Electr. Insul., Vol. 13, pp. 953-962, 2006.[5]Z. Hu and H. v. Seggern, ”Breakdown-induced polarization buildup in porous fluoropolymer sandwiches: a thermally stable piezoelectret“, J. Appl. Phys. 99, 024102, 2006.[6]J. Hillenbrand and G. M. Sessler, “Piezoelektret-Richtmikrofone auf Basis des Interferenzprinzips”, in Fortschritte der Akustik, Deutsche Arbeitsgemeinschaft für Akustik, DAGA ’06, pp. 59-60, 2006.[7]J. Hillenbrand, X. Zhang, and G. M. Sessler, “Piezoelektret-Mikrofone mit Fluorpolymerfolien und verbesserter Temperaturstabilität”, in Fortschritte der Akustik, Deutsche Arbeitsgemeinschaft für Akustik, DAGA ’07, pp. 495-496, 2007. [8]M. Tikander and H. Penttinen, “Sound quality differences between electret film (Emfit) and piezoelectric under-saddle guitar pickups“, 120th Audio Engineering Society (AES) Convention, Pre-print 6669, Paris, France, 2006.[9]A. Streicher, M. Kaltenbacher, R. Lerch, and H. Peremans, “Broadband EMFi ultrasonic transducers for bat research“, IEEE Ultrasonics Symposium, pp. 1629-1632, 2005.[10] J. L. Ealo, J. J. Camacho, and C. Fritsch, “Airborne ultrasonicphased arrays using ferroelectrets: A new fabrication approach”, IEEE Trans. Ultrason., Ferroel. and Freq. Control Vol. 56, pp. 848-858, 2009. [11] G. Gautschi, “Piezoelectric Sensorics”, Springer, Berlin, 2002 [12] S. P. Beeby, G. Ensel, M. Kraft, N. White, MEMS MechanicalSensors , Artech House, London, UK, 2004. [13] M. Kodejska, J. Hillenbrand, and G. M. Sessler, “Ferroelectret-filmaccelerometers with high sensitivities” in International Conference on Acoustics, Nederlands Akoestisch Genootschap / Deutsche Arbeitsgemeinschaft für Akustik, NAG / DAGA ’09, Deutsche Gesellschaft für Akustik, pp. 144-145, 2009. [14] X. Zhang, J. Hillenbrand, and G. M. Sessler, “Improvement ofpiezoelectric activity of cellular polymers by a double-expansion process”, J. Phys. D: Appl. Phys., Vol. 37, pp. 2146-2150, 2004. [15] X. Zhang, J. Hillenbrand, and G. M. Sessler, “Improvement ofpiezoelectric coefficient of cellular polypropylene films by repeated expansions”, J. Electrostat., Vol. 65, pp. 94-100, 2007. [16] J. Hillenbrand, X. Zhang, J. Zhang, and G. M. Sessler, “Pressure-treated cellular polypropylene with large piezoelectric coefficients”, IEEE Conf. Electr. Insul. Dielectr. Phenomena., pp. 40-43, 2003. [17] J. Hillenbrand and G. M. Sessler, “DC-biased ferroelectrets withlarge piezoelectric d 33-coefficients”, J. Appl. Phys. Vol. 103, 074103, 2008. [18] J. Hillenbrand, G. M. Sessler, and X. Zhang, "Verification of amodel for the piezoelectric d 33-coefficient of cellular electret films", J. Appl. Phys. Vol. 98, 064105, 2005.Joachim Hillenbrand received the diploma degree in physics from the University of Ulm, Germany, in 1992 and the Ph.D. degree from the Technical University of Darmstadt, Germany, in 1997. From 1993 to 1997 he worked at the German heavy ion research center GSI, Darmstadt, in the material research group. In 1998 he developed video- and audio-software at AIST, Ampfing, Germany. In 1999 he joined the electroacoustics group of theUniversity of Darmstadt. His research interests areelectrets, piezoelectric polymers and the application of these materials in the acoustic field.Miloš Kodejška was born in July 1983 in the Czech Republic. He received the M.Sc. degree in mechatronics from the Technical University of Liberec in 2007. Now he is studying Ph.D. course at the Institute of Mechatronics and Computer Engineering at the Faculty of Mechatronics, Informatics and Interdisciplinary Sciences, Technical University of Liberec.Yann Garcin was born in April 1985 in France. He received the double diploma degree in electronics and telecommunication from the School of Electronic Engineering ENSEA, France and the Technical University of Darmstadt, Germany, in 2009. His research concerns piezoelectric polymers, electrets and the application of these materials in accelerometers.Heinz von Seggern studied physics at the University of Goettingen and Hannover in the field of solid state physics where he graduated in 1976. He received the Ph.D. degree from the Technical University of Darmstadt in 1979, then became a Postdoc and later a principal investigator at Bell Laboratories. In 1982 his work was honoured by the Physics Prize of the Academy of Science of Goettingen. In 1985 he accepted a position as principal investigator atSiemens Research Center in Erlangen where he was promoted in 1990 to the position of department head. In 1997 he was selected as full professor at the Technical University of Darmstadt. His scientific interests are directed toward electrical properties of dielectrics, organic electronics (OFET, OLED) and towards medical imaging. He has published over 170 scientific papers in peer-reviewed journals.Gerhard M. Sessler (F’77) was born in Rosenfeld, Germany, on 15 February 1931. He studied physics at the Universities of Freiburg and Munich and received the Dr. rer. nat. degree in 1959 from the University of Goettingen. From 1959 to 1975, Sessler was a member of the Acoustics Research Department of Bell Laboratories where he had responsibilities for work on electret transducers, concert hall acoustics, and charge storage phenomena insolids. In 1975, he joined the University of Technology in Darmstadt as a professor of electroacoustics. He has since been involved in various fields of acoustics and solid-state physics, in particular in acoustic silicon transducers, in electret and piezoelectric materials, and in acoustic signal processing. Professor Sessler is an Inductee of the National Inventors Hall of Fame, a corresponding member of the Heidelberg Academy of Science, a Fellow or Member of various other professional societies in the USA andin Europe.。
DB11-806-2011 地面辐射供暖技术规范

本规范主要技术内容是以热水为热媒和以加热电缆为加热元 件的地面辐射供暖工程的应用技术,包括在北京地区已推广应 用、成熟可靠的新工艺;在国内或北京地区应用较少、积累经验 不够充分的工艺和材料暂未纳入。本技术规范共分6章:总则, 术语,设计,材料和设备,施工,检验、调试及验收。其中附录 A、附录 B、附录 D、 附 录 G、 附 录 H、 附 录 J、 附 录 K、 附 录 L、附录 M 为资料性附录,附录 C、附录 E、附录 F 为规范性附 录。
3
犇犅11/806-2011
目 次
1 总则 1 2 术语 2 3 设计 9 3.1 一般规定 9 3.2 地面构造 11 3.3 房间热负荷计算 14 3.4 地面散热量和系统供热量计算 15 3.5 热水系统设计 17 3.6 热 水 系 统 一 次 分 水 器 、 集 水 器 及 附 件 的 选 用 和 设 计 19 3.7 热水系统管道水力计算 20 3.8 加热电缆系统设计 22 3.9 热计量和室温控制 24 3.10 电气设计 25 4 材 料 28 4.1 一般规定 28 4.2 绝热层和预制沟槽保温板材料 28 4.3 填充层材料 31 4.4 热水系统的材料和设备 31 4.5 加热电缆材料和温控设备 33 5 施工 34 5.1 一般规定 34 5.2 发泡水泥绝热层的浇注 35 5.3 泡 沫 塑 料 类 绝 热 层 、 保 温 板 、 供 暖 板 及 其 填 充 板 的
本规范由北京市住房和城乡建设委员会、北京市质量技术监 督局负责管理,由主编单位负责具体技术内容的解释。在规范实 施过程中,如发现需要修改或补充之处,请将意见和建议反馈给 北京市建筑 设 计 研 究 院 (地 址: 北 京 市 南 礼 士 路 62 号, 邮 编: 100045) 北京市建设工程物资协会地暖分会 (地址:北京市西城 区 南 礼 士 路 头 条 3 号 , 电 话 :010-88070911, 邮 编 :100045)。
严重段塞流流型周期数学模型
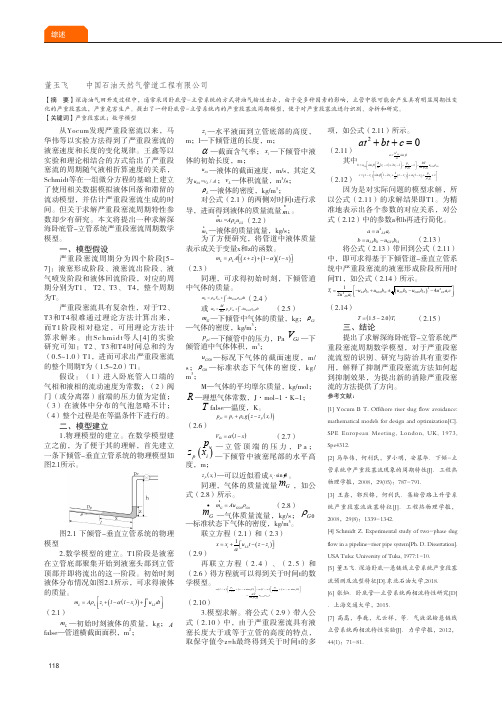
118从Yocum发现严重段塞流以来,马华伟等以实验方法得到了严重段塞流的液塞速度和长度的变化规律。
王鑫等以实验和理论相结合的方式给出了严重段塞流的周期随气液相折算速度的关系,Schmidt等在一组微分方程的基础上建立了使用相关数据模拟液体回落和滞留的流动模型,并估计严重段塞流生成的时间。
但关于求解严重段塞流周期特性参数却少有研究。
本文将提出一种求解深海卧底管-立管系统严重段塞流周期数学模型。
一、模型假设严重段塞流周期分为四个阶段[5-7]:液塞形成阶段、液塞流出阶段、液气喷发阶段和液体回流阶段,对应的周期分别为T1、 T2、T3、 T4,整个周期为T。
严重段塞流具有复杂性,对于T2、T3和T4很难通过理论方法计算出来,而T1阶段相对稳定,可用理论方法计算求解来。
由S c h mi d t 等人[4]的实验研究可知:T2、T3和T4时间总和约为(0.5~1.0)T1,进而可求出严重段塞流的整个周期T为(1.5~2.0)T1。
假设:(1)进入卧底管入口端的气相和液相的流动速度为常数;(2)阀门(或分离器)前端的压力值为定值;(3)在液体中分布的气泡忽略不计;(4)整个过程是在等温条件下进行的。
二、模型建立1.物理模型的建立。
在数学模型建立之前,为了便于其的理解,首先建立一条下倾管-垂直立管系统的物理模型如图2.1所示。
图2.1 下倾管-垂直立管系统的物理模型2.数学模型的建立。
T1阶段是液塞在立管底部聚集开始到液塞头部到立管顶部并即将流出的这一阶段。
初始时刻液体分布情况如图2.1所示,可求得液体的质量。
()()tL L i i LS m A z l l x u dt ρα =+−−+∫ (2.1)L m —初始时刻液体的质量,kg;A false—管道横截面面积,m 2;i z —水平液面到立管底部的高度,m;l—下倾管道的长度,m;α—截面含气率;i x —下倾管中液体的初始长度,m;LS u —液体的截面速度,m/s,其定义为=/LS L u v A ;L v —体积流量,m 3/s;L ρ—液体的密度,kg/m 3;对公式(2.1)的两侧对时间t进行求导,进而得到液体的质量流量L m •。
稳定分层湍流的PIV实验研究_邱翔
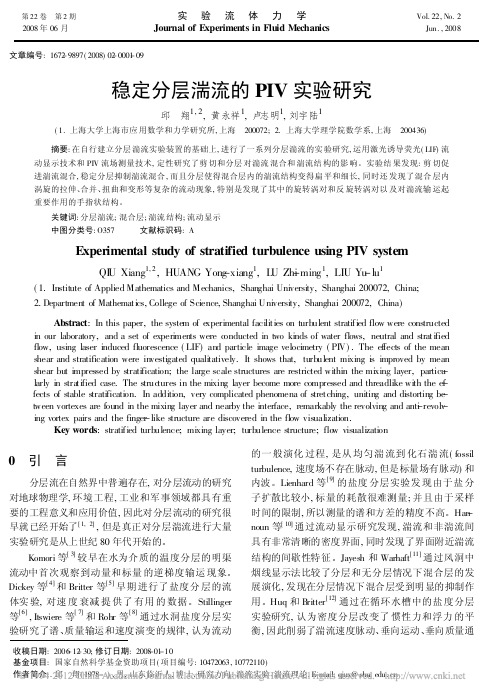
第22卷 第2期实验流体力学Vol.22,No.2 2008年06月Journal of Experiments in Fluid MechanicsJun.,2008文章编号:1672-9897(2008)02-0001-09稳定分层湍流的PIV 实验研究邱 翔1,2,黄永祥1,卢志明1,刘宇陆1(1.上海大学上海市应用数学和力学研究所,上海 200072; 2.上海大学理学院数学系,上海 200436) 摘要:在自行建立分层湍流实验装置的基础上,进行了一系列分层湍流的实验研究,运用激光诱导荧光(LIF)流动显示技术和PIV 流场测量技术,定性研究了剪切和分层对湍流混合和湍流结构的影响。
实验结果发现:剪切促进湍流混合,稳定分层抑制湍流混合,而且分层使得混合层内的湍流结构变得扁平和细长,同时还发现了混合层内涡旋的拉伸、合并、扭曲和变形等复杂的流动现象,特别是发现了其中的旋转涡对和反旋转涡对以及对湍流输运起重要作用的手指状结构。
关键词:分层湍流;混合层;湍流结构;流动显示 中图分类号:O357 文献标识码:AExperimental study of stratified turbulence using PIV systemQI U Xiang 1,2,HUANG Yong -xiang 1,L U Zh-i ming 1,LIU Yu -lu1(1.Institute of Applied Mathematics and Mechanics,Shanghai University,Shanghai 200072,China;2.Department of Mathematics,College of Science,Shanghai University,Shanghai 200072,China)Abstract :In this paper,the syste m of e xperimental facilities on turbulent stratified flow were constructed in our laboratory,and a set of experiments were c onducted in two kinds of water flows,neutral and stratified flow,using laser induced fluorescence (LIF)and particle image velocimetry (PI V).The effects of the mean shear and stratification were investigated qualitatively.It shows that,turbulent mixing is improved by mean shear but impressed by stratification;the large scale structures are restricted within the mixing layer,particu -larly in stra tified case.The struc tures in the mixing layer become more compressed and threadlike with the ef -fects of stable stratification.In addition,very complicated phenomena of stretching,uniting and distorting be -tween vortexes are found in the mixing layer and nearby the interface,remarkably the revolving and ant-i revolv -ing vortex pairs and the finger -like structure are discovered in the flow visualization.Key words :stratified turbulence;mixing layer;turbulence structure;flo w visualization收稿日期:2006-12-30;修订日期:2008-01-10基金项目:国家自然科学基金资助项目(项目编号:10472063,10772110)作者简介:邱 翔(1978-),男,山东临沂人,博士.研究方向:湍流实验、湍流理论.E -mail:qiux@0 引 言分层流在自然界中普遍存在,对分层流动的研究对地球物理学,环境工程,工业和军事领域都具有重要的工程意义和应用价值,因此对分层流动的研究很早就已经开始了[1,2],但是真正对分层湍流进行大量实验研究是从上世纪80年代开始的。
氧化镁的导热系数
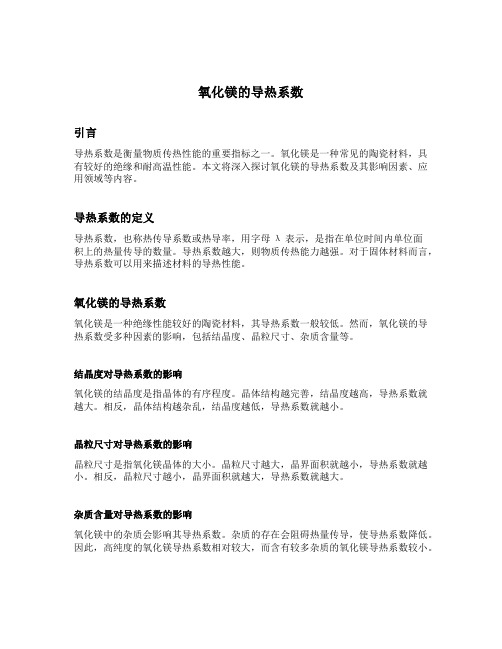
氧化镁的导热系数引言导热系数是衡量物质传热性能的重要指标之一。
氧化镁是一种常见的陶瓷材料,具有较好的绝缘和耐高温性能。
本文将深入探讨氧化镁的导热系数及其影响因素、应用领域等内容。
导热系数的定义导热系数,也称热传导系数或热导率,用字母λ表示,是指在单位时间内单位面积上的热量传导的数量。
导热系数越大,则物质传热能力越强。
对于固体材料而言,导热系数可以用来描述材料的导热性能。
氧化镁的导热系数氧化镁是一种绝缘性能较好的陶瓷材料,其导热系数一般较低。
然而,氧化镁的导热系数受多种因素的影响,包括结晶度、晶粒尺寸、杂质含量等。
结晶度对导热系数的影响氧化镁的结晶度是指晶体的有序程度。
晶体结构越完善,结晶度越高,导热系数就越大。
相反,晶体结构越杂乱,结晶度越低,导热系数就越小。
晶粒尺寸对导热系数的影响晶粒尺寸是指氧化镁晶体的大小。
晶粒尺寸越大,晶界面积就越小,导热系数就越小。
相反,晶粒尺寸越小,晶界面积就越大,导热系数就越大。
杂质含量对导热系数的影响氧化镁中的杂质会影响其导热系数。
杂质的存在会阻碍热量传导,使导热系数降低。
因此,高纯度的氧化镁导热系数相对较大,而含有较多杂质的氧化镁导热系数较小。
氧化镁导热系数的应用由于氧化镁的导热系数较低,因此其在绝缘材料领域有广泛的应用。
以下是氧化镁导热系数应用的几个例子:1. 电子器件绝缘氧化镁具有较好的绝缘性能,适用于电子器件的绝缘材料。
其低导热系数有助于减少电子器件中的热量损失。
2. 高温绝缘由于氧化镁具有良好的耐高温性能,适用于高温绝缘领域。
其低导热系数能够有效地减少热量的传导,保护设备和材料。
3. 锅炉和炉膛绝缘氧化镁可用作锅炉和炉膛的绝缘材料。
其低导热系数能够减少能量的损失,提高锅炉和炉膛的效率。
4. 太阳能热集热器绝缘太阳能热集热器需要一种绝缘材料来减少热量的损失。
氧化镁的低导热系数使其成为太阳能热集热器的理想绝缘材料之一。
总结本文综述了氧化镁的导热系数及其影响因素、应用领域等内容。
-196- +100℃高低温摄像装置低温性能实验研究
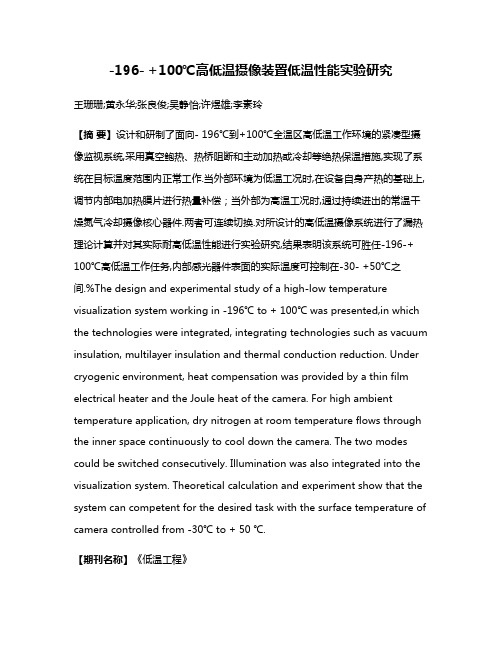
-196- +100℃高低温摄像装置低温性能实验研究王珊珊;黄永华;张良俊;吴静怡;许煜雄;李素玲【摘要】设计和研制了面向- 196℃到+100℃全温区高低温工作环境的紧凑型摄像监视系统,采用真空鲍热、热桥阻断和主动加热或冷却等绝热保温措施,实现了系统在目标温度范围内正常工作.当外部环境为低温工况时,在设备自身产热的基础上,调节内部电加热膜片进行热量补偿;当外部为高温工况时,通过持续进出的常温干燥氮气冷却摄像核心器件.两者可连续切换.对所设计的高低温摄像系统进行了漏热理论计算并对其实际耐高低温性能进行实验研究,结果表明该系统可胜任-196-+ 100℃高低温工作任务,内部感光器件表面的实际温度可控制在-30- +50℃之间.%The design and experimental study of a high-low temperature visualization system working in -196℃ to + 100℃ was presented,in which the technologies were integrated, integrating technologies such as vacuum insulation, multilayer insulation and thermal conduction reduction. Under cryogenic environment, heat compensation was provided by a thin film electrical heater and the Joule heat of the camera. For high ambient temperature application, dry nitrogen at room temperature flows through the inner space continuously to cool down the camera. The two modes could be switched consecutively. Illumination was also integrated into the visualization system. Theoretical calculation and experiment show that the system can competent for the desired task with the surface temperature of camera controlled from -30℃ to + 50 ℃.【期刊名称】《低温工程》【年(卷),期】2012(000)003【总页数】5页(P5-9)【关键词】高低温;摄像监视系统;绝热措施;空间环境模拟;低温医学应用【作者】王珊珊;黄永华;张良俊;吴静怡;许煜雄;李素玲【作者单位】上海交通大学制冷与低温工程研究所上海200240;上海交通大学制冷与低温工程研究所上海200240;上海交通大学制冷与低温工程研究所上海200240;上海交通大学制冷与低温工程研究所上海200240;上海交通大学制冷与低温工程研究所上海200240;上海交通大学制冷与低温工程研究所上海200240【正文语种】中文【中图分类】TB6631 引言由于最终要暴露在太空恶劣的环境中长期工作,经历剧烈的冷热交变冲击,航天装置被发射升空前需在地面环境模拟实验室内进行运动性能测试。