Exploration of Antifouling Potential of the Brown
反重力研究英语
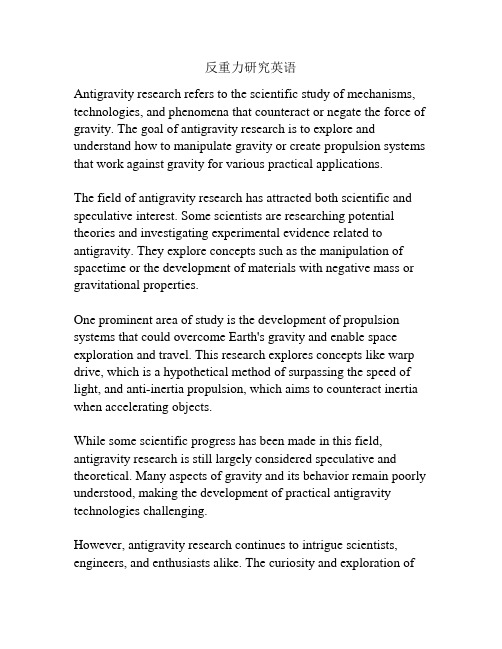
反重力研究英语Antigravity research refers to the scientific study of mechanisms, technologies, and phenomena that counteract or negate the force of gravity. The goal of antigravity research is to explore and understand how to manipulate gravity or create propulsion systems that work against gravity for various practical applications.The field of antigravity research has attracted both scientific and speculative interest. Some scientists are researching potential theories and investigating experimental evidence related to antigravity. They explore concepts such as the manipulation of spacetime or the development of materials with negative mass or gravitational properties.One prominent area of study is the development of propulsion systems that could overcome Earth's gravity and enable space exploration and travel. This research explores concepts like warp drive, which is a hypothetical method of surpassing the speed of light, and anti-inertia propulsion, which aims to counteract inertia when accelerating objects.While some scientific progress has been made in this field, antigravity research is still largely considered speculative and theoretical. Many aspects of gravity and its behavior remain poorly understood, making the development of practical antigravity technologies challenging.However, antigravity research continues to intrigue scientists, engineers, and enthusiasts alike. The curiosity and exploration ofsuch concepts push the boundaries of our understanding and may potentially lead to breakthroughs in the future.。
海洋探索重要的原因英语作文
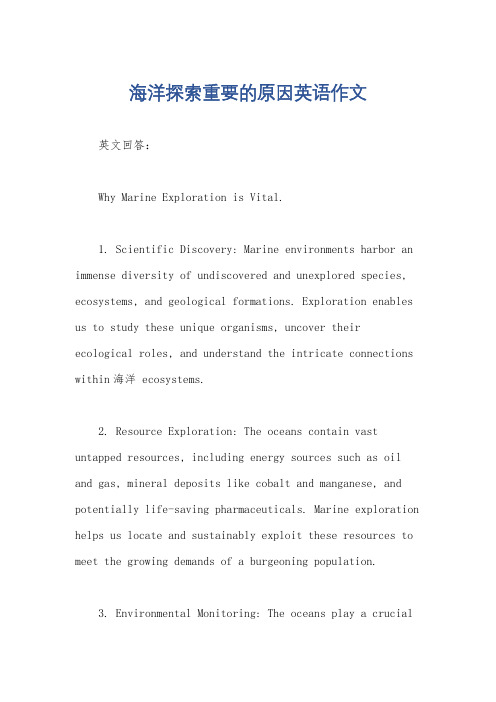
海洋探索重要的原因英语作文英文回答:Why Marine Exploration is Vital.1. Scientific Discovery: Marine environments harbor an immense diversity of undiscovered and unexplored species, ecosystems, and geological formations. Exploration enables us to study these unique organisms, uncover their ecological roles, and understand the intricate connections within海洋 ecosystems.2. Resource Exploration: The oceans contain vast untapped resources, including energy sources such as oil and gas, mineral deposits like cobalt and manganese, and potentially life-saving pharmaceuticals. Marine exploration helps us locate and sustainably exploit these resources to meet the growing demands of a burgeoning population.3. Environmental Monitoring: The oceans play a crucialrole in regulating the Earth's climate and supporting life on the planet. Marine exploration allows us to monitor ocean health, track pollution levels, and identify areas of environmental vulnerability. By understanding these processes, we can develop strategies for protecting and conserving marine ecosystems for future generations.4. Technological Innovation: Marine exploration drives technological advancements that have far-reaching applications. Specialized equipment, sensors, and submersibles developed for underwater exploration have led to breakthroughs in imaging, robotics, and navigation. These innovations not only benefit marine science but also have applications in other fields such as healthcare, manufacturing, and defense.5. Education and Inspiration: Marine exploration captures the imagination of people worldwide, inspiring a sense of wonder and curiosity about the unknown. By sharing discoveries and engaging in outreach programs, marine explorers educate the public about the importance of ocean conservation and foster a passion for STEM fields.6. Economic Development: Marine exploration creates economic opportunities through tourism, recreational activities, and the development of marine-based industries. By attracting visitors, researchers, and businesses, marine exploration contributes to local economies and promotes sustainable development in coastal communities.7. Political and Diplomatic Collaboration: Marine exploration often involves international cooperation and collaboration, fostering diplomatic relationships and promoting global cooperation on ocean governance. By addressing transnational challenges such as pollution and climate change, marine exploration strengthensinternational partnerships and contributes to global stability.中文回答:海洋探索的重要性。
猜猜是什么动物的英语作文

猜猜是什么动物的英语作文Guess What AnimalThe animal kingdom is a vast and diverse realm, home to an incredible array of creatures that captivate our imaginations and inspire our curiosity. From the majestic predators that rule the wilderness to the delicate and intricate insects that pollinate our flowers, each species possesses its own unique characteristics and adaptations that make it a remarkable part of the natural world. Today, we embark on a journey to explore the mysteries of one such animal, challenging you to guess what it is.Let's begin by painting a vivid picture of this enigmatic creature. Imagine a being that seamlessly blends into its surroundings, its very existence a testament to the wonders of camouflage. This animal possesses a remarkable ability to change its appearance, seamlessly shifting its hues and patterns to match the environment it inhabits. Whether it's the lush foliage of a rainforest, the rugged terrain of a desert, or the harsh, icy landscapes of the Arctic, this creature can adapt and conceal itself with unparalleled skill.As you ponder the clues, consider the diverse habitats that this animal might call home. Is it a creature that thrives in the vast, open savannas of Africa, where it must evade the watchful eyes of its predators? Or perhaps it resides in the dense, tangled jungles of South America, where it can use its camouflage to ambush unsuspecting prey? The possibilities are endless, and the more you delve into the details, the closer you may come to solving the mystery.One of the most captivating aspects of this animal is its unique method of locomotion. Imagine a being that can move with such grace and agility, seemingly defying the laws of physics as it navigates through its environment. This creature might possess the ability to climb vertical surfaces with ease, scurrying up trees or scaling rocky cliffs with the same fluidity as it traverses the ground. Its movements are a testament to the incredible adaptations that have evolved over millennia, allowing it to thrive in a wide range of habitats.As you contemplate the various possibilities, consider the sensory abilities of this enigmatic creature. Does it possess keen eyesight, allowing it to detect the slightest movement in its surroundings? Or perhaps it has a highly developed sense of smell, enabling it to track its prey or detect the presence of potential mates. The way in whichthis animal perceives and interacts with its world is a crucial piece of the puzzle, and understanding these capabilities can bring us closer to identifying it.Another intriguing aspect of this animal is its social behavior. Does it live a solitary life, prowling the wilderness alone in search of sustenance? Or does it thrive in close-knit family groups, relying on the strength and cohesion of its community to ensure its survival? The way in which this creature navigates the complex social dynamics of the animal kingdom can provide valuable insights into its overall nature and the strategies it employs to thrive.As you delve deeper into the clues, consider the unique adaptations that this animal may possess. Does it have specialized features that allow it to excel in a particular environment or to fulfill a specific ecological role? Perhaps it has a remarkable defense mechanism, such as the ability to release a foul-smelling secretion or to play dead in the face of danger. These adaptations are often the key to understanding the unique evolutionary journey of a species and the challenges it has overcome to survive.Ultimately, the identity of this captivating creature remains a mystery, waiting to be unraveled by your keen powers of observation and deduction. As you ponder the clues and weigh the possibilities, remember that the natural world is a tapestry of wonders, eachspecies a masterpiece of evolution and adaptation. By exploring the unique characteristics and behaviors of this enigmatic animal, you not only uncover its identity but also gain a deeper appreciation for the incredible diversity of life that surrounds us.So, what do you think? Can you guess what animal this is? The answer may surprise you, but the journey of discovery is sure to be an exhilarating one. Dive in, explore the clues, and let your curiosity be your guide as you unravel the secrets of this remarkable creature.。
不同浓度氯化钾对小鼠海马神经元膜电位的影响
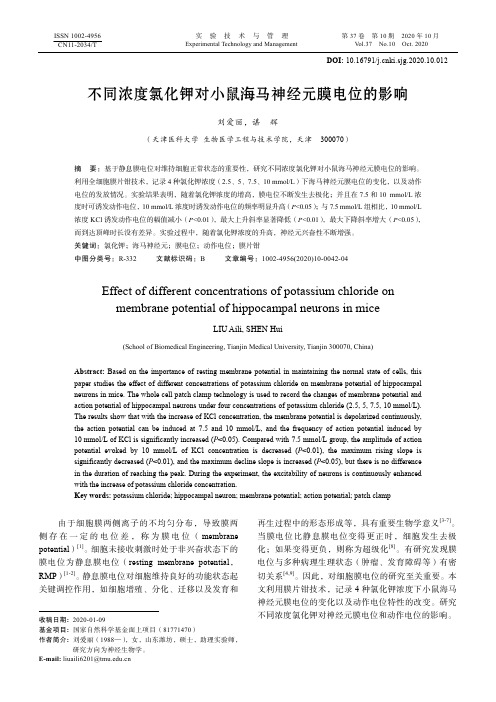
实 验 技 术 与 管 理 第37卷 第10期 2020年10月Experimental Technology and Management Vol.37 No.10 Oct. 2020ISSN 1002-4956 CN11-2034/TDOI: 10.16791/ki.sjg.2020.10.012不同浓度氯化钾对小鼠海马神经元膜电位的影响刘爱丽,谌 辉(天津医科大学 生物医学工程与技术学院,天津 300070)摘 要:基于静息膜电位对维持细胞正常状态的重要性,研究不同浓度氯化钾对小鼠海马神经元膜电位的影响。
利用全细胞膜片钳技术,记录4种氯化钾浓度(2.5、5、7.5、10 mmol/L )下海马神经元膜电位的变化,以及动作电位的发放情况。
实验结果表明,随着氯化钾浓度的增高,膜电位不断发生去极化;并且在7.5和10 mmol/L 浓度时可诱发动作电位,10 mmol/L 浓度时诱发动作电位的频率明显升高(P <0.05);与7.5 mmol/L 组相比,10 mmol/L 浓度KCl 诱发动作电位的幅值减小(P <0.01),最大上升斜率显著降低(P <0.01),最大下降斜率增大(P <0.05),而到达顶峰时长没有差异。
实验过程中,随着氯化钾浓度的升高,神经元兴奋性不断增强。
关键词:氯化钾;海马神经元;膜电位;动作电位;膜片钳中图分类号:R-332 文献标识码:B 文章编号:1002-4956(2020)10-0042-04Effect of different concentrations of potassium chloride onmembrane potential of hippocampal neurons in miceLIU Aili, SHEN Hui(School of Biomedical Engineering, Tianjin Medical University, Tianjin 300070, China)Abstract: Based on the importance of resting membrane potential in maintaining the normal state of cells, this paper studies the effect of different concentrations of potassium chloride on membrane potential of hippocampal neurons in mice. The whole cell patch clamp technology is used to record the changes of membrane potential and action potential of hippocampal neurons under four concentrations of potassium chloride (2.5, 5, 7.5, 10 mmol/L). The results show that with the increase of KCl concentration, the membrane potential is depolarized continuously, the action potential can be induced at 7.5 and 10 mmol/L, and the frequency of action potential induced by 10 mmol/L of KCl is significantly increased (P <0.05). Compared with 7.5 mmol/L group, the amplitude of action potential evoked by 10 mmol/L of KCl concentration is decreased (P <0.01), the maximum rising slope is significantly decreased (P <0.01), and the maximum decline slope is increased (P <0.05), but there is no difference in the duration of reaching the peak. During the experiment, the excitability of neurons is continuously enhanced with the increase of potassium chloride concentration.Key words: potassium chloride; hippocampal neuron; membrane potential; action potential; patch clamp由于细胞膜两侧离子的不均匀分布,导致膜两侧存在一定的电位差,称为膜电位(membrane potential )[1]。
Exploring the Frontiers of Biology
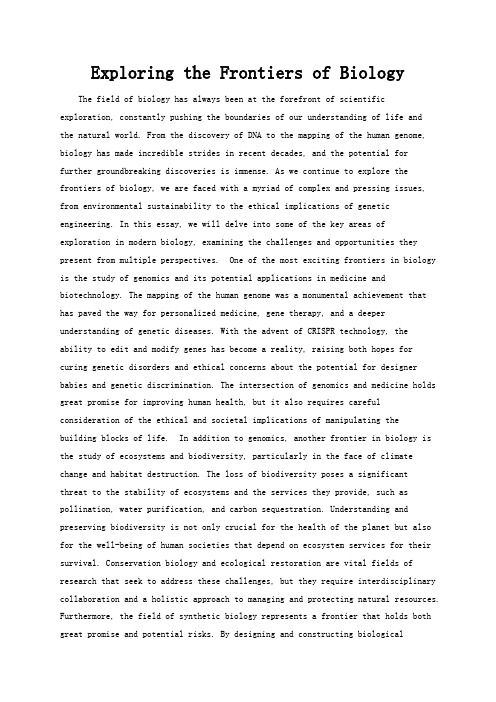
Exploring the Frontiers of Biology The field of biology has always been at the forefront of scientificexploration, constantly pushing the boundaries of our understanding of life andthe natural world. From the discovery of DNA to the mapping of the human genome, biology has made incredible strides in recent decades, and the potential forfurther groundbreaking discoveries is immense. As we continue to explore the frontiers of biology, we are faced with a myriad of complex and pressing issues, from environmental sustainability to the ethical implications of genetic engineering. In this essay, we will delve into some of the key areas ofexploration in modern biology, examining the challenges and opportunities they present from multiple perspectives. One of the most exciting frontiers in biologyis the study of genomics and its potential applications in medicine and biotechnology. The mapping of the human genome was a monumental achievement thathas paved the way for personalized medicine, gene therapy, and a deeper understanding of genetic diseases. With the advent of CRISPR technology, theability to edit and modify genes has become a reality, raising both hopes forcuring genetic disorders and ethical concerns about the potential for designer babies and genetic discrimination. The intersection of genomics and medicine holds great promise for improving human health, but it also requires carefulconsideration of the ethical and societal implications of manipulating thebuilding blocks of life. In addition to genomics, another frontier in biology is the study of ecosystems and biodiversity, particularly in the face of climate change and habitat destruction. The loss of biodiversity poses a significantthreat to the stability of ecosystems and the services they provide, such as pollination, water purification, and carbon sequestration. Understanding and preserving biodiversity is not only crucial for the health of the planet but alsofor the well-being of human societies that depend on ecosystem services for their survival. Conservation biology and ecological restoration are vital fields of research that seek to address these challenges, but they require interdisciplinary collaboration and a holistic approach to managing and protecting natural resources. Furthermore, the field of synthetic biology represents a frontier that holds both great promise and potential risks. By designing and constructing biologicalsystems and organisms, synthetic biology has the potential to revolutionize industries such as medicine, agriculture, and energy production. However, the deliberate engineering of living organisms also raises concerns about biosecurity, environmental impact, and unintended consequences. The development of biocontainment strategies and rigorous ethical guidelines is essential to ensure the responsible advancement of synthetic biology, balancing innovation with the precautionary principle to minimize potential harm. Moreover, the exploration of the microbiome, the diverse community of microorganisms that inhabit the human body and the environment, has opened up new avenues for understanding health and disease. The microbiome plays a crucial role in digestion, immunity, and even mental health, and research in this field has the potential to lead to new therapies and interventions. However, the complexity of the microbiome and its interactions with the host organism present significant challenges in deciphering its functions and developing targeted interventions. The study of the microbiome exemplifies the intricate and interconnected nature of biological systems, highlighting the need for interdisciplinary approaches and a deep appreciation of the complexity of life. Additionally, the ethical implications of biological research and its applications cannot be overlooked. As we venture into uncharted territories in genomics, synthetic biology, and other cutting-edge fields, we are confronted with ethical dilemmas that demand careful consideration and public dialogue. Questions about privacy, consent, equity, and the potential misuse of biotechnologies require thoughtful reflection and robust ethical frameworks to guide responsible innovation. Engaging diverse stakeholders, including scientists, policymakers, ethicists, and the public, is essential to ensure that the benefits of biological research are equitably distributed and that the risks are mitigated. In conclusion, the frontiers of biology present us with unprecedentedopportunities to advance our understanding of life and to harness its potentialfor the betterment of humanity and the planet. However, these frontiers also pose complex challenges that demand interdisciplinary collaboration, ethical reflection, and a deep sense of responsibility. As we continue to explore the frontiers of biology, we must strive to balance scientific innovation with ethical considerations, environmental stewardship, and societal well-being. By embracing aholistic and inclusive approach to biological research, we can navigate these frontiers with wisdom and humility, ensuring that our exploration of the natural world leads to sustainable and equitable outcomes for all.。
研究外太空的作文英语
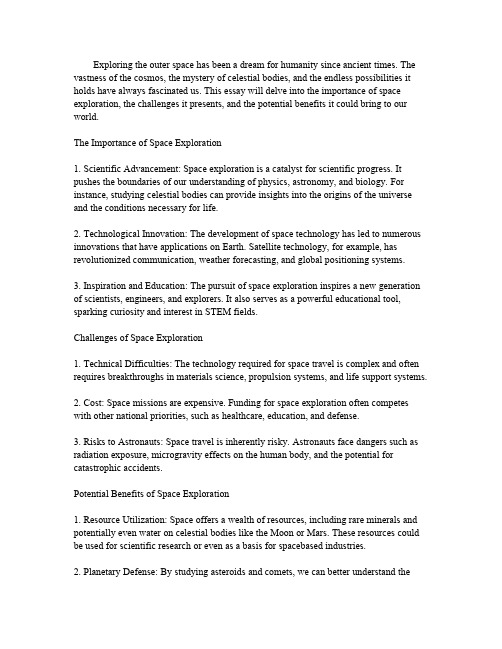
Exploring the outer space has been a dream for humanity since ancient times.The vastness of the cosmos,the mystery of celestial bodies,and the endless possibilities it holds have always fascinated us.This essay will delve into the importance of space exploration,the challenges it presents,and the potential benefits it could bring to our world.The Importance of Space Exploration1.Scientific Advancement:Space exploration is a catalyst for scientific progress.It pushes the boundaries of our understanding of physics,astronomy,and biology.For instance,studying celestial bodies can provide insights into the origins of the universe and the conditions necessary for life.2.Technological Innovation:The development of space technology has led to numerous innovations that have applications on Earth.Satellite technology,for example,has revolutionized communication,weather forecasting,and global positioning systems.3.Inspiration and Education:The pursuit of space exploration inspires a new generation of scientists,engineers,and explorers.It also serves as a powerful educational tool, sparking curiosity and interest in STEM fields.Challenges of Space Exploration1.Technical Difficulties:The technology required for space travel is complex and often requires breakthroughs in materials science,propulsion systems,and life support systems.2.Cost:Space missions are expensive.Funding for space exploration often competes with other national priorities,such as healthcare,education,and defense.3.Risks to Astronauts:Space travel is inherently risky.Astronauts face dangers such as radiation exposure,microgravity effects on the human body,and the potential for catastrophic accidents.Potential Benefits of Space Exploration1.Resource Utilization:Space offers a wealth of resources,including rare minerals and potentially even water on celestial bodies like the Moon or Mars.These resources could be used for scientific research or even as a basis for spacebased industries.2.Planetary Defense:By studying asteroids and comets,we can better understand therisks they pose to Earth and develop strategies to mitigate potential impacts.3.Search for Extraterrestrial Life:One of the most compelling reasons for exploring space is the possibility of discovering extraterrestrial life.This could fundamentally change our understanding of biology and our place in the universe.4.Longterm Survival of Humanity:Establishing a human presence beyond Earth could be crucial for the longterm survival of our species.As Earth faces environmental challenges and potential catastrophic events,having a backup in space could ensure the continuation of human civilization.ConclusionWhile the challenges of space exploration are significant,the potential benefits are immense.As we continue to push the boundaries of our knowledge and capabilities,the cosmos offers a frontier of discovery that could lead to a better understanding of our universe and our place within it.The pursuit of space exploration is not just about reaching for the stars it is about the growth and survival of humanity itself.。
干旱对藻造成的氧化应激
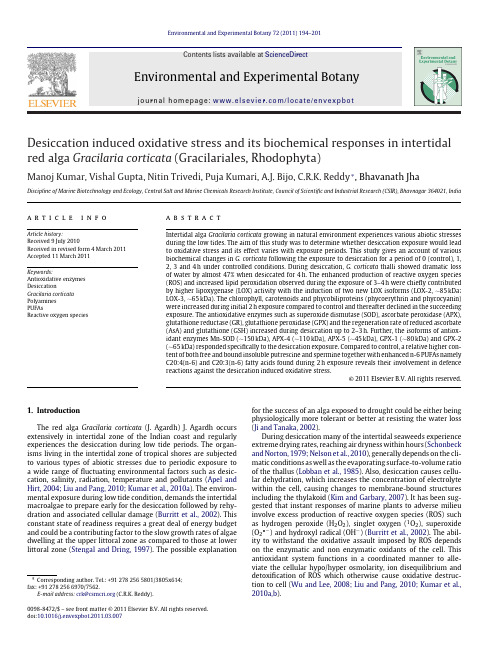
Environmental and Experimental Botany 72 (2011) 194–201Contents lists available at ScienceDirectEnvironmental and ExperimentalBotanyj o u r n a l h o m e p a g e :w w w.e l s e v i e r.c o m /l o c a t e /e n v e x p b otDesiccation induced oxidative stress and its biochemical responses in intertidal red alga Gracilaria corticata (Gracilariales,Rhodophyta)Manoj Kumar,Vishal Gupta,Nitin Trivedi,Puja Kumari,A.J.Bijo,C.R.K.Reddy ∗,Bhavanath JhaDiscipline of Marine Biotechnology and Ecology,Central Salt and Marine Chemicals Research Institute,Council of Scientific and Industrial Research (CSIR),Bhavnagar 364021,Indiaa r t i c l e i n f o Article history:Received 9July 2010Received in revised form 4March 2011Accepted 11March 2011Keywords:Antioxidative enzymes DesiccationGracilaria corticata Polyamines PUFAsReactive oxygen speciesa b s t r a c tIntertidal alga Gracilaria corticata growing in natural environment experiences various abiotic stresses during the low tides.The aim of this study was to determine whether desiccation exposure would lead to oxidative stress and its effect varies with exposure periods.This study gives an account of various biochemical changes in G.corticata following the exposure to desiccation for a period of 0(control),1,2,3and 4h under controlled conditions.During desiccation,G.corticata thalli showed dramatic loss of water by almost 47%when desiccated for 4h.The enhanced production of reactive oxygen species (ROS)and increased lipid peroxidation observed during the exposure of 3–4h were chiefly contributed by higher lipoxygenase (LOX)activity with the induction of two new LOX isoforms (LOX-2,∼85kDa;LOX-3,∼65kDa).The chlorophyll,carotenoids and phycobiliproteins (phycoerythrin and phycocyanin)were increased during initial 2h exposure compared to control and thereafter declined in the succeeding exposure.The antioxidative enzymes such as superoxide dismutase (SOD),ascorbate peroxidase (APX),glutathione reductase (GR),glutathione peroxidase (GPX)and the regeneration rate of reduced ascorbate (AsA)and glutathione (GSH)increased during desiccation up to 2–3h.Further,the isoforms of antiox-idant enzymes Mn-SOD (∼150kDa),APX-4(∼110kDa),APX-5(∼45kDa),GPX-1(∼80kDa)and GPX-2(∼65kDa)responded specifically to the desiccation pared to control,a relative higher con-tent of both free and bound insoluble putrescine and spermine together with enhanced n-6PUFAs namely C20:4(n-6)and C20:3(n-6)fatty acids found during 2h exposure reveals their involvement in defence reactions against the desiccation induced oxidative stress.© 2011 Elsevier B.V. All rights reserved.1.IntroductionThe red alga Gracilaria corticata (J.Agardh)J.Agardh occurs extensively in intertidal zone of the Indian coast and regularly experiences the desiccation during low tide periods.The organ-isms living in the intertidal zone of tropical shores are subjected to various types of abiotic stresses due to periodic exposure to a wide range of fluctuating environmental factors such as desic-cation,salinity,radiation,temperature and pollutants (Apel and Hirt,2004;Liu and Pang,2010;Kumar et al.,2010a ).The environ-mental exposure during low tide condition,demands the intertidal macroalgae to prepare early for the desiccation followed by rehy-dration and associated cellular damage (Burritt et al.,2002).This constant state of readiness requires a great deal of energy budget and could be a contributing factor to the slow growth rates of algae dwelling at the upper littoral zone as compared to those at lower littoral zone (Stengal and Dring,1997).The possible explanation∗Corresponding author.Tel.:+912782565801/3805x614;fax:+912782566970/7562.E-mail address:crk@ (C.R.K.Reddy).for the success of an alga exposed to drought could be either being physiologically more tolerant or better at resisting the water loss (Ji and Tanaka,2002).During desiccation many of the intertidal seaweeds experience extreme drying rates,reaching air dryness within hours (Schonbeck and Norton,1979;Nelson et al.,2010),generally depends on the cli-matic conditions as well as the evaporating surface-to-volume ratio of the thallus (Lobban et al.,1985).Also,desiccation causes cellu-lar dehydration,which increases the concentration of electrolyte within the cell,causing changes to membrane-bound structures including the thylakoid (Kim and Garbary,2007).It has been sug-gested that instant responses of marine plants to adverse milieu involve excess production of reactive oxygen species (ROS)such as hydrogen peroxide (H 2O 2),singlet oxygen (1O 2),superoxide (O 2•−)and hydroxyl radical (OH −)(Burritt et al.,2002).The abil-ity to withstand the oxidative assault imposed by ROS depends on the enzymatic and non enzymatic oxidants of the cell.This antioxidant system functions in a coordinated manner to alle-viate the cellular hypo/hyper osmolarity,ion disequilibrium and detoxification of ROS which otherwise cause oxidative destruc-tion to cell (Wu and Lee,2008;Liu and Pang,2010;Kumar et al.,2010a,b ).0098-8472/$–see front matter © 2011 Elsevier B.V. All rights reserved.doi:10.1016/j.envexpbot.2011.03.007M.Kumar et al./Environmental and Experimental Botany72 (2011) 194–201195Conversely,in the recent years ROS have shown to have the mul-tifaceted roles in signalling and stress responses(Foyer and Noctor, 2005)as it can also generate oxygenated polyunsaturated fatty acids(Ox-PUFAs)defending the oxidative stress.A great deal of information supporting the involvement of Ox-PUFAs in abiotic and biotic stresses has also recently implicated the function of lipoxy-genase(LOX)enzyme in the stress physiology(Maksymiec and Krupa,2006;Rucinska and Gwozdz,2005).Ritter et al.(2008)also reported the synthesis of octadecanoid and eicosanoid oxygenated derivatives in Laminaria digitata following the exposure to Cu stress. Further,polyamines(PAs)–aliphatic amines with relatively low molecular mass have been studied in macroalgae for the matu-ration of reproductive structure in Grateloupia(Sacramento et al., 2007)and Gracilaria cornea(Guzman-Uriostegui et al.,2002).Most recently,Kumar et al.(2010a)explained the potential defensive role of polyamines particularly putrescine and of PUFAs especially n-6PUFAs(C18:3(n-6)and C18:2(n-6))under oxidative stress con-ditions.It is thus conceivable that polyamines and PUFAs could be the robust effectors for scavenging the ROS and thus counteract the desiccation stress.Although,the recovery of physiological processes(photosyn-thesis and nutrient uptake)following the desiccation has been studied extensively(Kim et al.,2009;Abe et al.,2001;Ji and Tanaka,2002),little information is available on the cellular mech-anisms that operate within the intertidal seaweeds to mitigate the oxidative stress arising from desiccation(Burritt et al.,2002; Sampath-Wiley et al.,2008).In view of this,we hypothesized that enzymatic and non enzymatic antioxidants,PUFAs and endogenous polyamines may play a crucial role in minimizing the ROS gener-ation under desiccation stress.Thus,wefirstly estimated the lipid peroxidation,ROS generation and their subsequent histochemical localization as an evidence of cellular damage as function of des-iccation exposure.Subsequently,the changes in the isoforms of major antioxidative enzymes,LOX and endogenous accumulation of polyamines were examined to understand how these different systems regulate in a coherent manner as a means of desiccation tolerance.2.Materials and methods2.1.Algal cultureThe vegetative thalli of G.corticata were collected from inter-tidal region during low tide periods from Veraval Coast(20◦54 N, 70◦22 E),Gujarat,India.Selected clean and young thalli were then brought to the laboratory in a cool pack.In order to ini-tiate unialgal culture,the fronds were cleaned manually with brush in autoclaved seawater to remove epiphytic foreign mat-ters.The fronds thus cleaned were acclimatized to laboratory conditions in aerated cultures in PES medium(Provasoli,1968) supplemented with germanium dioxide(5mg L−1)for10d.Dur-ing the acclimatization period,the medium was replenished twice at5d interval and maintained under white coolfluorescent tube lights at50mol photons m−2s−1with a12:12h light:dark cycle at22±1◦C.2.2.Desiccation treatment and determination of relative water contentIn the control treatment,the thalli were maintained under submerged conditions in seawater at the culture conditions as described above.For desiccation treatment the thalli were exposed to the air while keeping their bottom ends(∼1cm)in the wet sand spread on a propylene plastic tray at25±2◦C with relative humid-ity65±2%in culture room.The experiments were set up with three replicates and were measured at0(control),1,2,3,4and5h for all biochemical parameters.The relative water content(RWC)after the exposure was calcu-lated as follows:RWC=(LW t−DW)/(FW−DW)×100,where LW t is the weight of the thalli subjected to the desiccation treatment for t hours,dry weight(DW)is the weight of the thalli oven dried for 48h at80◦C,and FW is the weight of the thalli before desiccation.2.3.Determination of lipid peroxidation and ROSThe level of lipid peroxidation in the thallus was determined as described by Heath and Packer(1968).Tissue(0.2g)was extracted in2mL of0.5%thiobarbituric acid(TBA)prepared in20%trichloro acetic acid(TCA).Extract was heated at95◦C for30min and then quickly cooled on ice.After centrifugation at10,000×g for10min, the absorbance of the supernatant was measured at532nm.Cor-rection of non-specific turbidity was made by subtracting the absorbance value taken at600nm.The level of lipid peroxidation was expressed as nmol of malondialdehyde(MDA)formed using an extinction coefficient of155mM cm−1.ROS were determined according to the procedure described by Contreras et al.(2005). Individuals of G.corticata(1g FW)incubated for1h at15◦C in 100mL of5M2,4-dichlorofluoresceine diacetate(Calbiochem, San Diego,CA,USA)dissolved infiltered seawater.After incuba-tion,the tissue was rinsed in seawater,blotted dry,weighed,and frozen in liquid nitrogen.The tissue was then ground in liquid nitrogen,suspended in5mL of40mM Tris–HCl buffer,pH7.0,and centrifuged at15,000×g for25min.Fluorescence was determined using LS-5spectro-fluorometer(Perkin-Elmer,Norwalk,CT,USA)at an excitation wavelength of488nm and at an emission wavelength of525nm.Fluorescence values were obtained using a standard curve of2,4-dichlorofluoresceine(DCF;Sigma,St.Louis,MO,USA).2.4.Histochemical detection of O2•−and H2O2The production of O2•−and H2O2in response to desiccation was analysed according to Castro-Mercado et al.(2009).For the detection of O2•−radicals the hand cut sections of control and des-iccated thalli(20in number)were immersed in5mL detection solution containing0.05%nitroblue tetrazolium(NBT)in50mM potassium phosphate buffer(pH6.4)and10mM NaN3.The sec-tions were infiltrated under vacuum for3min in the same solution and illuminated for2h until appearance of dark spots,character-istic of blue formazan precipitates.Stained sections were cleared by boiling in acetic:glycerol:ethanol(1:1:3,v/v/v)solution before photographs were taken.H2O2production was visually detected by an endogenous peroxidase-dependent staining procedure using 3,3 -diaminobenzidine(DAB).Hand cut sections of control and des-iccated thalli(20in number each)were immersed in DAB solution 1mg/mL(pH5.0),vacuum-infiltrated for5min and then incubated at room temperature for8h in the presence of light till brown spots appeared.Sectioned were bleached by immersing in boiling ethanol to visualize the brown spots and photographs were taken.To ver-ify the specificity of precipitates,before staining with DAB some sections were immersed for2h in solution containing the H2O2 scavenger1mM ascorbate(data not shown).2.5.Determination of photosynthetic pigmentsThe photosynthetic pigments were estimated by following the method of Dawes et al.(1999).Chlorophyll,carotenoids(80% acetone)and phycobiliproteins(100mM phosphate buffer,pH 6.5)were extracted by grinding the sample(1g FW)in respec-tive solutions(1:3,w/v)in the dark and cold conditions followed by centrifugation at3000×g at4◦C for15min.Absorbance was recorded at662and645for chlorophyll,470nm for carotenoids196M.Kumar et al./Environmental and Experimental Botany72 (2011) 194–201and620,650,and565nm for phycobiliproteins by using Shi-madzu UV spectrophotometer(UV-160,Shimadzu,Japan).The amount of chlorophyll and carotenoids pigments was calculated according to Lichtentaler and Wellburn(1985).Phycoerythrin(PE), phycocyanin(PC)and allophycocyanin(APC)contents were esti-mated using the equations described by Tandeau and Houmard (1988).2.6.Determination of antioxidative enzymes,water soluble antioxidants,lipoxygenase and polyaminesThe activities of antioxidant enzymes namely superoxide dis-mutase(SOD),ascorbate peroxidase(APX),glutathione reductase (GR)and glutathione peroxidase(GPX)and water soluble antiox-idants were determined as described by Contreras et al.(2005). Extract for LOX was prepared according to the method of Tsai et al. (2008)and assayed by measuring the increase in absorbance at 234nm with substrate linolenic acid(100M)prepared in ethanol. The increase in absorbance due to the accumulation of conjugate dienes was monitored at234nm for10min.The activity was cal-culated using the extinction coefficient of conjugated dienes(ε, 25mM−1cm−1).Polyamines were extracted in5%TCA following the method described by Guzman-Uriostegui et al.(2002).TCA extracts were analysed for free polyamines following dansylation in a mixture consisted of200L TCA extract,400L dansyl chloride (Sigma,5mg mL−1in acetone),and200L saturated Na2CO3.After incubation at70C for1h,100L proline(100mg mL−1)was added to remove excess dansyl chloride and extracted in0.5mL of ethyl acetate by vortexing for15–30s.The organic layer containing the polyamines was separated by low speed centrifugation(3000×g), removed and dried under nitrogen.An aliquote of50L was used for spotting onto LK60Whatman silica gel thin layer chromatog-raphy(TLC)plates.Identification of polyamines was accomplished by comparison of R f values with authentic polyamine standards (Put,Spd and Spm obtained from Sigma as hydrochlorides)on TLC using the solvent system:cyclohexane:ethylacetate(5:4,v/v) and chloroform:triethylamine(25:2,v/v).Dansyl-polyamines were scrapped off from the TLC plates and redissolved in600L ace-tone.The samples thus obtained were shaken and centrifuged at6000×g for3min,and500L of this solution was quan-tified using high-resolution spectro-fluorometer(Perkin-Elmer, Norwalk,CT,USA)with excitation at365nm and emission at 510nm.2.7.Activity staining of antioxidative enzymes and lipoxygenaseThe isoenzyme profile of antioxidative enzyme and lipoxy-genase was determined using10%or12%non-denaturating polyacrylamide gels using their specific activity staining proce-dures for SOD(Beauchamp and Fridovich,1971),APX(Mittler and Zilinskas,1994),GPX(Lin et al.,2002)and LOX(Heinisch et al., 1996).The molecular mass of enzyme isoforms was evaluated comparing with the standard molecular weight marker contain-ing myosin,210kDa;-galactosidase,135kDa;bovine serum albumin,80kDa;soyabean trypsin inhibitor,31.5kDa;lysozyme, 18.2kDa.2.8.Determination of fatty acidsFatty acids from lipids were converted to respective methyl esters according to Kumar et al.(2010b)by trans-methylation using 1%NaOH in methanol and heated for15min at55◦C,followed by the addition of5%methanolic HCl and again heated for15min at 55◦C.Fatty acid methyl esters(FAMEs)were extracted in hexane and analysed by GC-2010coupled with GCMS-QP2010.3.Statistical analysisResults are expressed as mean of three replicates with standard deviation.Statistical analyses were performed by one way analysis of variance(ANOVA).Significant differences between means were tested by the least significant difference(LSD)at0.05probability levels.4.Results4.1.Relative water content,lipid peroxidation and ROSDesiccation exposure caused a significant decrease in the RWC that was contingent to exposure duration and decreased by almost 47%and58%(p<0.05)in the thalli desiccated for4h and5h respec-tively compared to control.The thalli desiccated for5h were not able to survive when rehydrated back in natural seawater for24h. Therefore,the thalli were exposed for a maximum of4h for sub-sequent experiments.The decrease in the RWC observed in the present study was accompanied with the accumulation of TBARS and ROS generation in the thalli(Table1).A threefold increase (p<0.05)in their content was found in the thalli exposed for3–4h. In view of this,a histochemical staining was employed to detect in situ accumulation of O2•−and H2O2radicals(two important representatives for ROS)using NBT and DAB respectively as the staining reagents(supplementary Fig.1).A blue formazone formed by reduction of NBT by O2•−is clearly evident for the generation of superoxide radical that appearedfirst in the epidermal cells (2h)then gradually progressed to cortical cells(3h)and later dis-tributed all over the tissue with the advancement of desiccation (4h)(supplementary Fig.1A).Similarly,the formation of H2O2 dependent brown precipitates was contingent with the exposure duration(supplementary Fig.1B)and accumulated maximum in the thalli exposed for longer duration(4h)where it drenched completely in to the epidermal as well as cortical cells.However, a short term desiccation did not produce detectable differences between control and thalli exposed for1h.Further,we also mea-sured the activity of LOX to ascertain its possible involvement in lipid peroxidation and generation of ROS.Desiccation exposure markedly enhanced the LOX activity(>3fold,p<0.05)in thalli exposed for3–4h(Table2)compared with the control activity (8.1nkatal min−1mg−1protein).In addition,prolonged exposure of 3–4h induced two new isoforms of LOX such as LOX-2(∼85kDa) and LOX-3(∼65kDa)in addition to LOX-1(∼110kDA)with a con-sistent increase in their intensity as the desiccation progress.4.2.Photosynthetic pigments,water soluble antioxidants and polyaminesA significant increase in chlorophyll and carotenoid concen-tration was observed during the initial exposure of2h with values80.48±3.44and38.93±4.49g g−1FW corresponded to an increase of almost1.5and1.75fold(p<0.05)respectively over the control values with53.32±3.94and22.32±4.38g g−1FW respectively.However,in the prolonged exposure of3–4h the con-tents of both were decreased gradually.Phycobiliproteins such as phycoerythrin(PE),phycocyanin(PC)and allophycocyanin(APC) showed varied responses with the desiccation exposure.The concentration of both PE and PC increased gradually with the des-iccation exposure and attained the peak values with565.81±19.88 and283.07±22.95g g−1FW after2h exposure.This corresponds to an increase of40%from the control amount with386.23±23.35 and201.33±11.56g g−1FW respectively.The degree of increase for APC following desiccation exposure was considerably low when compared with control.M.Kumar et al./Environmental and Experimental Botany72 (2011) 194–201197Table1Effect of desiccation exposure on relative water content,lipid peroxidation,reactive oxygen species and photosynthetic pigments of G.corticata(mean±SD,n=3). Parameter Desiccation hours(h)01234LSD(5%)RWC(%)100a82.64±2.76b70.66±3.09c58.90±2.09d52.69±2.59e 3.47 MDA(nmol g−1FW) 3.20±0.56d 4.63±0.57d 6.43±0.47c8.90±0.70b10.77±0.48a 2.10 ROS(mol g−1FW)0.17±0.03e0.32±0.04d0.47±0.05c0.66±0.07b0.80±0.05a0.08 Photosynthetic pigments( g g−1FW)Chlorophyll53.32±3.94c68.97±8.49b80.48±3.44a52.60±5.02c43.13±3.74d9.33 Carotenoids22.32±4.38cd30.17±2.21b38.93±4.49a24.61±2.73bc16.94±2.58d 6.21 Phycoerythrin386.23±23.35c475.50±15.87b565.81±19.88a412.38±34.85c315.18±8.39d40.53 Phycocyanin201.33±11.56b266.39±7.06a283.07±22.95a206.54±17.92b168.47±14.27c28.61 Allophycocyanin133.47±12.51b154.21±9.60a155.07±14.33134.10±7.77b127.56±13.84b15.89RWC,relative water content;MDA,malondialdehyde;ROS,reactive oxygen species;different superscript letters within row indicate significant differences at p<0.05 according to one way ANOVA.The contents of reduced(AsA),oxidized(DHA)and total (AsA+DHA)ascorbate were greatly influenced by desiccation expo-sure(Table2).The AsA showed a consistent higher amount throughout the experiment with the peak value2.41nmol g−1FW (2h)that correspond to an increase of2.4fold over the control value with1.04nmol g−1FW.DHA also gradually accumulated as the desiccation progress and increased to>2fold(p<0.05)after 4h when compared with control0.71nmol g−1FW.A significant accumulation of both AsA and DHA led to a remarkable increase in total ascorbate content with a maximum of3.87nmol g−1FW (2h)and was chiefly contributed by AsA.To determine the regen-eration of AsA,the ratio of AsA/DHA was calculated and found to increase only during the initial hours of exposure with peak ratio1.66(2h)compared to1.48(control)and decreased there-after till the end of experiment.The contents of reduced(GSH),total (GSH+GSSG)glutathione and their regeneration rate(GSH/GSSG) were quite higher(2.5–3fold,p<0.05)in the extended exposure of3h with values7.33,10.96and2.02nmol g−1FW respectively (Table2).The corresponding values in the control thalli were2.37, 4.32and1.22nmol g−1FW respectively.Among the polyamines such as putrescine(Put),spermidine (Spd)and spermine(Spm)investigated,Put was the predominant polyamine in all its three forms viz.free(F),bound soluble(BS) and bound insoluble(BI)followed by spermine and spermidine. In the control thalli,the accumulation of Put and Spm followed in the order of BS>BI>F and F>BS>BI respectively.Following the2h desiccation,the level of F-Put(43.01),BI-Put(41.05),F-Spm(22.04) and BI-Spm(7.61,1h)increased significantly(p<0.05)over the control values of14.02,33.42,12.10and1.07mol g−1FW respec-tively,and thereafter declined in the extended exposure(Table2). The content of BS-Spm increased by almost70%in short term des-iccation of less than2h over the control with9.30mol g−1FW, whereas BS-Put exhibited a constant decrease with the exposure duration.Table2Effect of desiccation exposure on enzymatic,water soluble antioxidants and polyamines in G.corticata(mean±SD,n=3).Parameter Desiccation hours(h)01234LSD(5%)Enzymes activity(U mg−1protein)SOD119.67±5.13c174.33±5.86b202.33±9.61a215.33±7.37a104.33±9.61d14.09 APX0.33±0.04d0.46±0.05c0.58±0.05b0.71±0.06a0.48±0.06c0.09GR0.52±0.03cd0.69±0.05c0.81±0.07b0.98±0.08a0.48±0.06d0.10 GPX0.63±0.06c0.60±0.04c0.92±0.06a0.76±0.05b0.53±0.06c0.09 LOX a8.10±0.62e12.81±1.22d18.74±1.45c25.12±3.22b31.02±3.11a 3.99 Antioxidants(nmol g−1FW)AsA 1.04±0.16c 1.61±0.12b 2.41±0.23a 1.87±0.22b 1.53±0.10b0.31 DHA0.71±0.08c 1.03±0.18c 1.46±0.13b 1.82±0.15ab 2.07±0.17a0.26AsA+DHA 1.76±0.15b 2.64±0.30b 3.87±0.34a 3.69±0.09a 3.60±0.12a0.40AsA/DHA 1.48±0.34ab 1.59±0.15a 1.66±0.08a 1.04±0.22bc0.74±0.10c0.30 GSH 2.37±0.25d 3.78±0.31c 5.51±0.30b7.33±0.30a 4.90±0.26b0.51 GSSG 1.95±0.03c 2.40±0.11c 2.76±0.50bc 3.63±0.24b 6.51±0.52a0.62 GSH+GSSG 4.32±0.27d 6.17±0.23c8.27±0.36b10.96±0.45a11.41±0.44a0.65 GSH/GSSG 1.22±0.11bc 1.58±0.20ab 2.05±0.49a 2.02±0.12a0.76±0.09c0.46 Polyamines( mol g−1FW)PutrescineF14.02±2.29d23.60±3.16c43.01±3.19a31.86±2.24b7.66±2.13d 4.81 BS46.85±6.01a13.73±2.97b36.98±3.58a17.14±2.01b9.19±1.67b 6.54 BI33.42±4.63bc55.47±3.72a41.05±3.65b26.95±3.07c 5.35±1.22d 6.28 SpermineF12.10±.18cd16.83±1.32b22.04±1.92a14.42±1.72bc9.55±1.65d 2.87 BS9.30±1.12bc15.78±1.94a13.30±1.06a9.85±1.04b 6.14±0.73c0.94 BI 1.07±0.10c7.61±1.01a 3.69±0.54b0.85±0.10d0.68±0.07d 2.26 SpermidineF 3.05±0.23b 2.13±0.26c 1.71±0.14cd 1.20±0.12d 6.10±0.36a0.43BS 3.94±0.28a 1.24±0.17b 1.12±0.07bc0.75±0.08c0.72±0.09c0.28 BI7.88±0.93a 6.08±0.43b7.02±0.39ab 4.28±0.56c 4.47±0.13c 1.00SOD,superoxide dismutase;APX,ascorbate peroxidase;GR,glutathione reductase;GPX,glutathione peroxidase;LOX,lipoxygenase;AsA and GSH,reduced ascorbate and glutathione respectively;DHA and GSSG,oxidized ascorbate and glutathione respectively;F,free;BI,bound soluble;BI,bound insoluble;different letters within row indicate significant differences at p<0.05according to one way ANOVA.a The unit of LOX is nkatal mg−1protein.198M.Kumar et al./Environmental and Experimental Botany72 (2011) 194–2014.3.Antioxidative enzymes and their isoformsSOD,APX,GR and GPX were selected as biomarkers to deter-mine the oxidative stress resulted from desiccation.All the studied enzymes exhibited enhanced activities duringfirst3h of exposure and thereafter reduced markedly.The specific activities of SOD,APX and GR increased by1.5–2.5fold(3h)with values215,0.71and 0.98U mg−1protein respectively over the control activities with 119,0.33and0.52U mg−1protein respectively(Table2).Thalli exposed for1h did not show any significant increase in GPX activ-ity,although gained the peak activity(0.92U mg−1protein)when exposed for2–3h and declined subsequently.Native PAGE sup-ported the spectrophotometric measurements of these enzymes (supplementary Fig.2).Apparent higher activity of SOD observed in the thalli throughout the experiment was solely attributed to Mn-SOD(∼150kDa)(supplementary Fig.2B)confirmed by using H2O2/KCN as inhibitors.APX in particular revealed the specific response to the desiccation exposure and exhibited three isoforms APX-1(∼125kDa),APX-2(∼85kDa),APX-3(∼60kDa)in control and1h desiccated thalli(supplementary Fig.2A).An additional isoform APX-4(∼110)exhibited in the samples exposed for2–4h. Further,the prolong exposure of3–4h is evident for the induction of APX-5(∼45kDa)with the complete inhibition of APX-3.The activ-ity gel of GPX(supplementary Fig.2C)displayed three GPX isoforms (GPX-1,2and3)with higher activity of GPX-3(∼35kDa)particu-larly in the control samples.The other isoforms GPX-1(∼80kDa) and GPX-2(∼65kDa)showed higher activity in the thalli exposed for2–3h while diminished in the samples desiccated for4h.4.4.Fatty acidsTable3summarizes the variation in fatty acid composition of G. corticata in response to desiccation stress.Palmitic(C16:0),stearic (C18:0),palmitoleic(C16:1,n-7),oleic(C18:1,n-9cis),linoleic (C18:2,n-6),dihomo-␥-linolenic(C20-3,n-6)and arachidonic acids (C20:4,n-6)were the foremost fatty acids that showed majorfluc-tuations following desiccation.The palmitic and arachidonic acid showed a reverse trend for their accumulation with the advance-ment of desiccation.The content of palmitic acid when decreased to minimum value19.88%TFA(2h)and corresponded to a signif-icant reduction of43%(p<0.01)over the control(34.83%TFA),a parallel increase of28%(2h)in the content of arachidonic acid was observed over the control52.54%TFA.During the prolong expo-sure a dramatic increase in palmitic acid(40.11%TFA,4h)was accompanied with a significant decline in arachidonic acid(46.98% TFA,4h).Among the other fatty acids,a substantial decrease in C18:0,C16:1(n-7),C18:1(n-9)trans and C20:5(n-3)fatty acids was analogized by a constant increase in C18:1(n-9)cis acid through-out the experiment.Interestingly,fatty acid C20:2(n-6)showed its existence only after an exposure of2h.The arachidonic and dihomo-␥-linolenic were the major polyunsaturated fatty acids contributed to almost58%TFA in control,while their level elevated to74%after an exposure of2h followed by a considerable decrease till the last sampling.Also,with the advancement of desiccation the ratio of UFA/SFA varied with a maximum of3.72(2h)and minimum 1.37(4h)compared with the control1.68.5.DiscussionAmong the variety of physical and biological factors determining algal zonation in intertidal region,desiccation tolerance/resistance also plays a decisive role in species distribution.The data obtained in the present study are clearly evident for the narrow tolerance competency of G.corticata and can tolerate the water loss of only 25–35%before it succumbs to desiccation stress.The water loss beyond35%(3–4h exposure)caused greater lipid peroxidation and accumulation of O2•−and H2O2radicals is evidenced by histochem-ical studies(supplementary Fig.1),confirming the state of oxidative stress.Superoxide radicals inactivate several enzymes important in energy production and amino acid metabolism.These enzymes have iron–sulfur clusters and their inactivation is caused by oxida-tion of the cluster,leading to release of iron,followed by Fenton chemistry.If O2•−levels rise(e.g.during desiccation),inactivation rates accelerate,repair cannot keep up and metabolic pathways are inhibited(Halliwell,2006).The role of H2O2in stress-induced dam-age has long been recognized,but it is now also generally accepted that H2O2is an integral component of cell signalling cascades and an indispensable secondary messenger in biotic and abiotic stress situations(Foyer and Noctor,2005).The results of ourfindings for desiccation tolerance are similar to Kim and Garbary(2007)who reported20–24%of water loss in Codium fragile.In contrary,Ji and Tanaka(2002)observed only13%water loss in Hizikia fusiformis and Gloipeltis furcata under2h of controlled desiccation.The intertidal Porphyra haitanensis could withstand a30%loss of water before major reduction in photosynthetic activity occurs(Zou and Gao, 2002).The water loss from thallus generally depends on the plant cell wall matrix composition,and surface texture(Moore et al., 2008).The volatile aldehyde like MDA and specific LOX isoen-zymes are suitable markers for membrane lipid peroxidation.As compared with control,the thalli desiccated for3–4h showed con-siderably higher lipid peroxidation and has positively correlated with enhanced activity of LOX.This enzyme generates singlet oxy-gen and superoxide anions while incorporating molecular oxygen in to linoleic and linolenic fatty acids,to form lipid hydroper-oxides.In the present study,the enhanced LOX activity together with the induction of two new isoform LOX-2and LOX-3in the thalli desiccated for3–4h could be attributed to the decreased arachidonic level which had been utilized as the substrate for its catalytic reaction.Moreover,the induced LOX isoforms could also be categorized in to type II lipoxygenases,as these isoforms were induced in the later hours of desiccation exposure when both chlorophyll and carotenoids got diminished.The type II lipoxyge-nases are widespread in plants,have a neutral pH-optimum and a strong tendency to show co-oxidation reactions(e.g.of chloro-phyll,carotenoids,and lipophilic vitamins)caused by free radicals liberated during the catalytic process(Siedow,1991).Recently, Contreras et al.(2009)described the induction of an arachidonic acid dependent LOX activity and its role in lipoperoxide produc-tion in Lessonia nigrecens and Scytosiphon lomentaria under copper exposure.The initial rise in the photosynthetic pigments during short term desiccation of2h suggests the greater demand for increased ATP (formed via cyclic photophosphorylation)for desiccation tolerance mechanism.Apparently,during this short term desiccation when the surface waterfilm gets evaporated,the atmospheric CO2can penetrate the cell and may enhance the photosynthesis(Zou and Gao,2002).But with the extended desiccation and more loss of water the electron system operating between photosystem II(PSII) and photosystem I(PSI)may get interrupted due to decreased photosynthetic pigments coupled with enhanced ROS.Moreover, a parallel increase in carotenoids during initial2h further sug-gests their role as an antioxidant by acting as physical quenchers of electronically exited molecules,in addition to functioning as photoreceptors(Woodall et al.,1997).Further,the increased phy-cobiliproteins particularly PE and PC evidenced their protective role under oxidative stress conditions.In red algae,phycobilipro-teins are synthesized in phycobilisomes and are associated with light harvesting centre(LHC)of PSII(Sampath-Wiley et al.,2008). Their increased level could be a possible acclamatory mechanism for G.corticata in response to desiccation induced oxidative stress as these proteins function as storage proteins for biosynthesis dur-。
保护探索海洋的四级英语作文

保护探索海洋的四级英语作文英文回答:Preserving the exploration of the ocean is crucial for the advancement of human knowledge and the well-being of our planet. By implementing comprehensive conservation measures and fostering sustainable practices, we can safeguard this vital realm for generations to come.First and foremost, establishing marine protected areas (MPAs) serves as a cornerstone in safeguarding the ocean's ecosystems. MPAs regulate human activities within designated areas, allowing for the recovery of depleted populations and the protection of biodiversity hot spots. By creating a safe haven for marine life, MPAs promote the replenishment of fish stocks, contribute to climate change mitigation, and enhance resilience to environmental stressors.Secondly, promoting sustainable fishing practices playsa pivotal role in preserving the health of marine ecosystems. Implementing responsible fishing techniques, such as the use of size-selective gears and adhering to catch limits, ensures the long-term viability of fish populations. Additionally, adopting ecosystem-based management approaches considers the interconnectedness of species and encourages holistic decision-making that minimizes ecological impacts.Furthermore, reducing plastic pollution is essentialfor protecting marine life. Plastic waste poses a significant threat to ocean health, entangling marine animals and leading to ingestion and starvation. Governments and industries must implement comprehensive waste management strategies, focusing on reducing the production and consumption of single-use plastics. Educational initiatives and public awareness campaigns can also contribute to changing consumer behavior and promoting responsible disposal practices.Finally, fostering international cooperation is paramount in addressing the global challenges facing theocean. Collaboration between nations enables the sharing of scientific knowledge, technological advancements, and best practices. By working together, countries can implement coordinated conservation measures, enforce regulations to prevent illegal fishing, and address transboundary issues that transcend national borders.In conclusion, protecting the exploration of the ocean requires a multifaceted approach that encompasses the establishment of marine protected areas, the promotion of sustainable fishing practices, the reduction of plastic pollution, and the fostering of international cooperation. By safeguarding this vast and vital realm, we ensure the health of marine ecosystems, the sustainable use of marine resources, and the advancement of scientific knowledge for future generations.中文回答:保护海洋探索对于人类知识的进步和我们星球的福祉至关重要。
青蒿素的发现对人类社会的重大意义英语作文
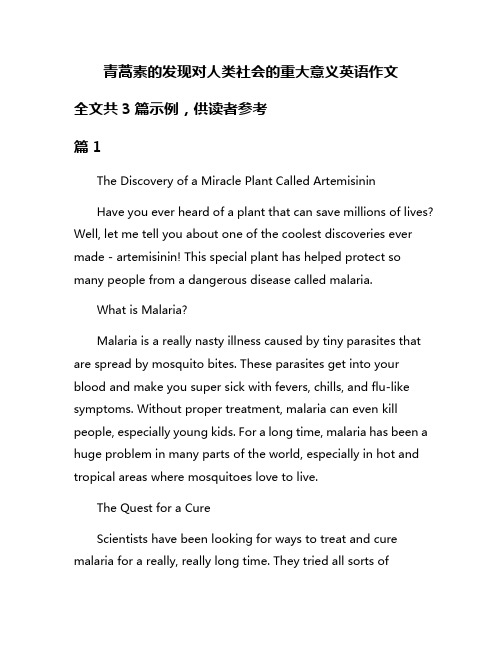
青蒿素的发现对人类社会的重大意义英语作文全文共3篇示例,供读者参考篇1The Discovery of a Miracle Plant Called ArtemisininHave you ever heard of a plant that can save millions of lives? Well, let me tell you about one of the coolest discoveries ever made - artemisinin! This special plant has helped protect so many people from a dangerous disease called malaria.What is Malaria?Malaria is a really nasty illness caused by tiny parasites that are spread by mosquito bites. These parasites get into your blood and make you super sick with fevers, chills, and flu-like symptoms. Without proper treatment, malaria can even kill people, especially young kids. For a long time, malaria has been a huge problem in many parts of the world, especially in hot and tropical areas where mosquitoes love to live.The Quest for a CureScientists have been looking for ways to treat and cure malaria for a really, really long time. They tried all sorts ofmedicines, but the malaria parasites kept becoming resistant, which means the medicines stopped working against them. It was like the parasites were playing a big game of "Can't catch me!" with the scientists and doctors.Enter ArtemisininThen, in the 1970s, something amazing happened. Chinese scientists discovered a plant called Artemisia annua, also known as sweet wormwood. This plant had been used in traditional Chinese medicine for centuries, but the scientists found out that it contained a special ingredient that could fight malaria like a superhero! They called this ingredient "artemisinin."Artemisinin turned out to be a game-changer in the battle against malaria. It was incredibly effective at killing the malaria parasites, and it worked in a way that made it really hard for the parasites to become resistant to it. It was like the scientists had finally found the kryptonite to the malaria parasites' superpowers!Saving Lives Around the WorldOnce artemisinin was discovered, scientists and doctors started using it to create new malaria treatments. These treatments have saved millions and millions of lives, especially inparts of the world where malaria is really common, like Africa, Asia, and South America.Just imagine how many kids, parents, grandparents, and friends have been able to stay healthy and happy because of this incredible plant! Children who might have died from malaria have grown up to become doctors, teachers, artists, and leaders, all thanks to artemisinin.A Gift from NatureWhat's even cooler is that artemisinin comes from a completely natural source – a plant! It just goes to show how amazing and powerful nature can be. For thousands of years, this special plant had been growing all around us, waiting to be discovered and used to help people.It's like nature left us a secret treasure, hidden in plain sight, just waiting for us to figure out its incredible powers. And when we did, it changed the world!The Nobel PrizeThe discovery of artemisinin was so important that in 2015, a scientist named Tu Youyou won the Nobel Prize for her work in finding and developing this lifesaving treatment. Can you imagine how proud she must have felt? She helped save millionsof lives just by studying a plant that had been growing all around us for centuries!It just goes to show that even the smallest things in nature can have huge impacts on the world. Who knows what other amazing secrets are hidden in the plants, animals, and environments around us, just waiting to be uncovered?A Brighter FutureThanks to artemisinin, the future looks a lot brighter for many people around the world who might have otherwise lost their lives to malaria. Kids can go to school, parents can work and take care of their families, and communities can thrive without the constant threat of this deadly disease.Of course, there's still more work to be done. Scientists are always looking for even better ways to treat and prevent malaria, and they're studying artemisinin to see if it can help with other diseases too. But for now, we can all be grateful for this incredible plant and the brilliant minds that discovered its lifesaving powers.So the next time you see a little green plant growing out of a crack in the sidewalk, remember the story of artemisinin. Who knows? Maybe that tiny sprout holds the secret to the next bigmedical breakthrough, just waiting to be found. Nature is full of amazing surprises, and the discovery of artemisinin proves that even the smallest things can change the world in the biggest ways.篇2The Awesome Discovery of Artemisinin and How it Helps People!Have you ever heard of a plant called sweet wormwood? It's a kind of herb that has bright yellow flowers. This plant might not look very special, but it actually contains something really amazing inside called artemisinin. Artemisinin is a super important chemical that helps cure a very bad disease called malaria.Malaria is a horrible sickness that is caused by tiny parasites called Plasmodium. These nasty parasites get inside people's bodies when they are bitten by infected mosquitoes. Once inside, the parasites start destroying red blood cells, which are the cells that carry oxygen around in your body. This can make people extremely sick with high fevers, chills, vomiting, and even organ failure if left untreated. Malaria is especially dangerous for youngkids and can sadly cause many deaths each year, especially in parts of Africa.For a very long time, people couldn't find a good cure for malaria. The medicines they tried didn't work well and the Plasmodium parasites kept developing resistance to the drugs. It was a terrible situation that left millions suffering. But then in the 1970s, an amazing scientist from China made an incredible discovery that changed everything!Her name was Tu Youyou, and she was researching traditional Chinese herbal medicines to find new treatments for malaria. After sorting through ancient texts and folk remedies, she came across sweet wormwood, which had been used for fevers for centuries. Tu Youyou realized this herb might be able to treat malaria too!Being a brilliant scientist, Tu Youyou spent years carefully extracting and testing the compounds from sweet wormwood. After so much hard work, she finally identified the special ingredient we now call artemisinin in 1972. When tested, artemisinin was incredibly effective at rapidly killing the malaria parasites!This discovery was groundbreaking. Artemisinin-based drugs became the best new weapons in treating malaria in manyyears. They worked better than older medicines with fewer side effects. Artemisinin combination therapies could clear infections quickly and reduce severe illness and deaths from malaria.Thanks to Tu Youyou's persistence and genius, artemisinin has saved millions of lives over the past decades! It's one of the greatest contributions to human health in the modern era. Artemisinin-based drugs are now used widely around the world, especially in developing countries hardest hit by malaria.For her remarkable work, Tu Youyou was honored with the Nobel Prize in Medicine in 2015. At age 84, she was the first Chinese Nobel laureate in medicine. Her inspirational story shows how diligence, intelligence, and care for humanity can lead to transformative discoveries that benefit people everywhere.The tale of artemisinin teaches us so much about human potential and our ability to overcome major challenges through science. With brilliant minds and dedicated researchers, we can develop innovative solutions to the biggest health issues facing the world today and in the future.Imagine all the other amazing plant-based cures that might still be awaiting discovery! Or cutting-edge treatments for other widespread diseases like cancer, HIV/AIDS, or Alzheimer'sdisease. Just like Tu Youyou unlocked the secrets of sweet wormwood, there could be other miracle medicines hidden in nature's pharmacy.As long as we have brave explorers willing to ask questions, scrutinize evidence, and endlessly pursue new knowledge, we can make the unknown known. Every scientific breakthrough, no matter how small, helps expand human understanding and our power to make life better for all people.So the next time you go outside and see a little herb or flower, remember how something as simple and unassuming as sweet wormwood led to one of the most important medical discoveries ever! With curiosity, hard work, and brilliant minds setting their focus, who knows what other miracles from nature await us to uncover next? The future is truly full of endless possibilities!篇3The Great Discovery of Artemisinin and Its Huge ImpactHave you ever heard of a plant called sweet wormwood? It's a type of mugwort that grows in many parts of Asia. This humble green plant with tiny yellow flowers doesn't look very special. But hidden inside its leaves is an amazing natural compound that hassaved millions of lives! I'm talking about artemisinin, a powerful drug that cures malaria.What is Malaria?Malaria is a terrible disease caused by a parasite that is transmitted by mosquitoes. When an infected mosquito bites you, it injects these malaria parasites into your blood. The parasites travel to your liver and multiply rapidly. A few days later, they invade your red blood cells and make you really sick with a high fever, chills, fatigue, vomiting, and other nasty symptoms.In severe cases, malaria can cause organ failure, seizures, coma, and even death. It's an especially big threat to young kids because their bodies aren't strong enough to fight it off. Millions of children die from malaria every year, mainly in parts of Africa, Asia, and South America where the disease is widespread. Just imagine how awful that must be for their families and communities.The Hunt for a CureFor a very long time, there was no good cure for malaria. The medicines used to treat it were expensive, hard to get, and didn't always work. The malaria parasite kept developing resistance toeach new drug. Scientists desperately searched for better remedies from plants and other natural sources.In the 1960s and 1970s, Chinese scientists made an incredibly important discovery. While studying ancient Chinese medical texts, they came across references to the sweet wormwood plant being used to treat fever-causing illnesses like malaria. Could this herb really hold the key to a powerful new malaria treatment after all?Tu Youyou and Her Team's PerseveranceThe scientist who led the search for antimalarial compounds from wormwood was a remarkable woman named Tu Youyou. Tu Youyou was born on December 30, 1930 on the island of Hangzhou near Shanghai. She had to overcome many challenges in her life just to get an education and become a researcher at a time when opportunities for women were very limited in China.But Tu Youyou was brilliant, hardworking and determined. She immersed herself in studying ancient medicinal texts and folk remedies. After many failed experiments, Tu's team finally discovered that a special extract from the wormwood plant could clear malaria parasites from the blood. This extract contained a miraculous compound called artemisinin.Artemisinin's Life-Saving ImpactArtemisinin turned out to be more effective against malaria than any medicine ever discovered! It worked quickly to stop malaria's devastating effects and had very few side effects. Tu Youyou's discovery helped launch a new era in finally controlling one of humanity's deadliest and most persistent diseases.Since the 1990s, medicines based on artemisinin have been widely used to treat hundreds of millions of malaria cases around the world. It is estimated that artemisinin combination therapies have saved over a million lives every year, the majority of them young children in poor countries. Just think about how many parents didn't have to suffer the horrible grief of losing their beloved sons and daughters to this evil illness, thanks to Tu Youyou's amazing work!Not only has artemisinin saved countless lives, it has also allowed many communities to grow and prosper rather than being constantly devastated by malaria's economic costs and productivity losses. Families could earn incomes, kids could go to school, and economies could develop when people were much healthier. Artemisinin has truly helped lift entire regions out of poverty and despair.A Nobel Prize for Tu YouyouFor her historic discovery that helped so many people, Tu Youyou was awarded the 2015 Nobel Prize in Physiology or Medicine, becoming the first Chinese woman to ever win a Nobel. Everyone celebrated her well-deserved achievement! Even though Tu was already 84 years old, she showed humility and gratitude, saying the real honor belonged to the countless scientists over centuries who contributed to the slow process of accumulating knowledge.Tu Youyou's life story of persistence and brilliant scientific deduction leading to a world-changing discovery is so inspiring! It just goes to show how perseverance, diligence, and careful investigation can allow anyone to make a difference, no matter how daunting the challenges. Her work reminds us of the importance of studying nature, tradition, and drawing wisdom from diverse sources.The hunt for new medicines from plants used in ancient folk remedies isn't over either. Who knows what other life-saving natural compounds are waiting to be discovered? Perhaps there is a student reading this essay who will become the next Tu Youyou and help solve another major disease that plagues humanity. The example of artemisinin proves we should never stop searching!。
探寻生态奥秘,保护自然环境作文
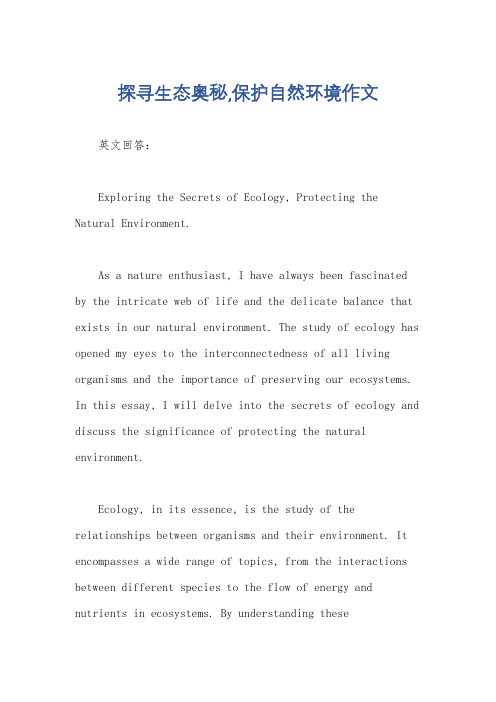
探寻生态奥秘,保护自然环境作文英文回答:Exploring the Secrets of Ecology, Protecting the Natural Environment.As a nature enthusiast, I have always been fascinated by the intricate web of life and the delicate balance that exists in our natural environment. The study of ecology has opened my eyes to the interconnectedness of all living organisms and the importance of preserving our ecosystems. In this essay, I will delve into the secrets of ecology and discuss the significance of protecting the natural environment.Ecology, in its essence, is the study of the relationships between organisms and their environment. It encompasses a wide range of topics, from the interactions between different species to the flow of energy and nutrients in ecosystems. By understanding theserelationships, we can gain insights into the functioning of ecosystems and the impact of human activities on the environment.One of the key concepts in ecology is the idea of biodiversity. Biodiversity refers to the variety of life forms present in a particular habitat or ecosystem. It includes not only the different species of plants and animals but also the genetic diversity within each species. Biodiversity is crucial for the stability and resilience of ecosystems. It provides a buffer against environmental disturbances and ensures the availability of essential ecosystem services, such as pollination and nutrient cycling.Unfortunately, human activities have led to a significant loss of biodiversity worldwide. Deforestation, habitat destruction, pollution, and climate change are some of the major threats to biodiversity. For instance, the destruction of tropical rainforests in the Amazon basin not only results in the loss of countless plant and animal species but also disrupts the intricate ecologicalinteractions that have evolved over millions of years.Protecting the natural environment is of paramount importance to ensure the survival of our planet and future generations. Conservation efforts can take various forms, from establishing protected areas to implementing sustainable resource management practices. For instance, the establishment of national parks and nature reserves helps to safeguard critical habitats and preserve biodiversity. These protected areas not only serve as havens for endangered species but also provideopportunities for ecotourism, which can contribute to local economies.In addition to protecting natural habitats, it is also essential to address the underlying causes of environmental degradation. This requires a shift in our mindset and behavior towards more sustainable practices. For example, reducing our reliance on fossil fuels and embracing renewable energy sources can help mitigate climate change and reduce air pollution. Similarly, adopting sustainable agricultural practices, such as organic farming andagroforestry, can promote soil health and biodiversity conservation.中文回答:生态奥秘的探索,保护自然环境。
保护臭氧英语作文
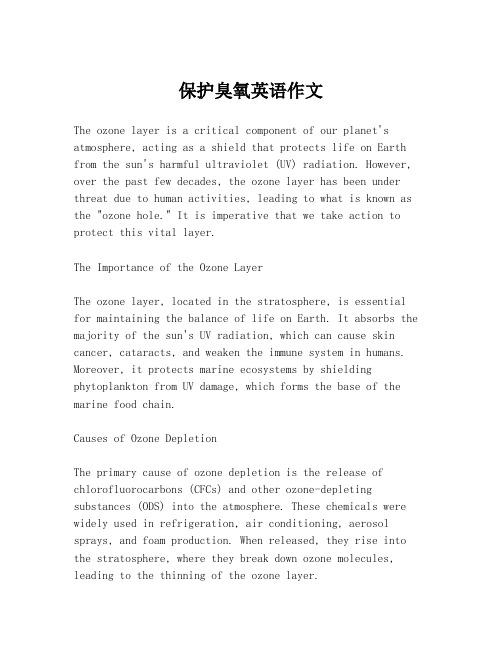
保护臭氧英语作文The ozone layer is a critical component of our planet's atmosphere, acting as a shield that protects life on Earth from the sun's harmful ultraviolet (UV) radiation. However, over the past few decades, the ozone layer has been under threat due to human activities, leading to what is known as the "ozone hole." It is imperative that we take action to protect this vital layer.The Importance of the Ozone LayerThe ozone layer, located in the stratosphere, is essential for maintaining the balance of life on Earth. It absorbs the majority of the sun's UV radiation, which can cause skin cancer, cataracts, and weaken the immune system in humans. Moreover, it protects marine ecosystems by shielding phytoplankton from UV damage, which forms the base of the marine food chain.Causes of Ozone DepletionThe primary cause of ozone depletion is the release of chlorofluorocarbons (CFCs) and other ozone-depleting substances (ODS) into the atmosphere. These chemicals were widely used in refrigeration, air conditioning, aerosol sprays, and foam production. When released, they rise into the stratosphere, where they break down ozone molecules, leading to the thinning of the ozone layer.International Efforts to Protect the Ozone LayerRecognizing the threat to the ozone layer, the international community came together to create the Montreal Protocol in 1987. This treaty aimed to phase out the production and consumption of CFCs and other ODS. The protocol has been successful in reducing the use of these substances, and as a result, the ozone layer is showing signs of recovery.Individual Actions to Protect the Ozone LayerWhile international agreements are crucial, individual actions also play a significant role in protecting the ozone layer. Here are some steps we can take:1. Reduce Energy Consumption: By conserving energy, we can reduce the demand for power, which often comes from sources that contribute to ozone depletion.2. Use Eco-Friendly Products: Opt for products that are free from ODS and support companies that are committed to sustainable practices.3. Educate Others: Spread awareness about the importance of the ozone layer and the actions that can be taken to protect it.4. Support Ozone-Friendly Technologies: Encourage the development and use of technologies that do not harm the ozone layer.ConclusionProtecting the ozone layer is a collective responsibility. It requires the concerted efforts of governments, businesses, and individuals. By taking proactive steps, we can ensurethat the ozone layer remains a robust shield against harmful UV radiation, safeguarding the health of our planet and its inhabitants for generations to come.。
有机氟化学 英文
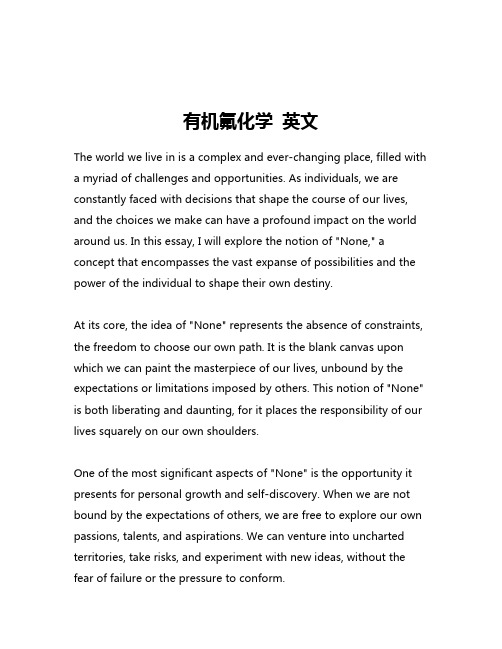
有机氟化学英文The world we live in is a complex and ever-changing place, filled with a myriad of challenges and opportunities. As individuals, we are constantly faced with decisions that shape the course of our lives, and the choices we make can have a profound impact on the world around us. In this essay, I will explore the notion of "None," a concept that encompasses the vast expanse of possibilities and the power of the individual to shape their own destiny.At its core, the idea of "None" represents the absence of constraints, the freedom to choose our own path. It is the blank canvas upon which we can paint the masterpiece of our lives, unbound by the expectations or limitations imposed by others. This notion of "None" is both liberating and daunting, for it places the responsibility of our lives squarely on our own shoulders.One of the most significant aspects of "None" is the opportunity it presents for personal growth and self-discovery. When we are not bound by the expectations of others, we are free to explore our own passions, talents, and aspirations. We can venture into uncharted territories, take risks, and experiment with new ideas, without the fear of failure or the pressure to conform.This freedom of choice, however, also comes with its own set of challenges. With the absence of a predetermined path, we must navigate the complexities of life with a keen sense of self-awareness and decision-making skills. We must be willing to confront our fears, overcome our doubts, and make tough choices that align with our values and aspirations.In this regard, the concept of "None" is inextricably linked to the notion of personal responsibility. When we embrace the idea of "None," we are taking ownership of our lives and the consequences of our actions. We must be willing to accept the successes and failures that come with our choices, and to learn from our experiences in order to grow and evolve.Moreover, the idea of "None" extends beyond the individual and into the realm of societal and global impact. When we recognize the power of our own choices and the potential to shape the world around us, we are empowered to make a difference. We can use our talents and resources to address pressing challenges, to advocate for change, and to inspire others to do the same.In this way, the concept of "None" is not just about personal freedom, but about the collective responsibility we have to one another and to the planet we share. By embracing the idea of"None," we can cultivate a sense of agency and empowerment that transcends our individual lives and contributes to the betterment of the world.Of course, the journey of "None" is not without its complexities and challenges. We may face obstacles, setbacks, and moments of uncertainty along the way. But it is precisely in these moments that the true power of "None" becomes evident. It is in our ability to adapt, to persevere, and to find new paths forward that we discover the true depth of our own resilience and the boundless potential of the human spirit.In conclusion, the concept of "None" is a powerful and multifaceted idea that challenges us to take ownership of our lives, to embrace the freedom of choice, and to make a meaningful impact on the world around us. It is a call to action, a invitation to explore the vast expanse of possibilities that lie before us, and to create a future that reflects our deepest values and aspirations. By embracing the idea of "None," we can unlock the true potential of the human experience and contribute to the betterment of our shared world.。
探索海洋可以帮助科学家发现新知识英语作文
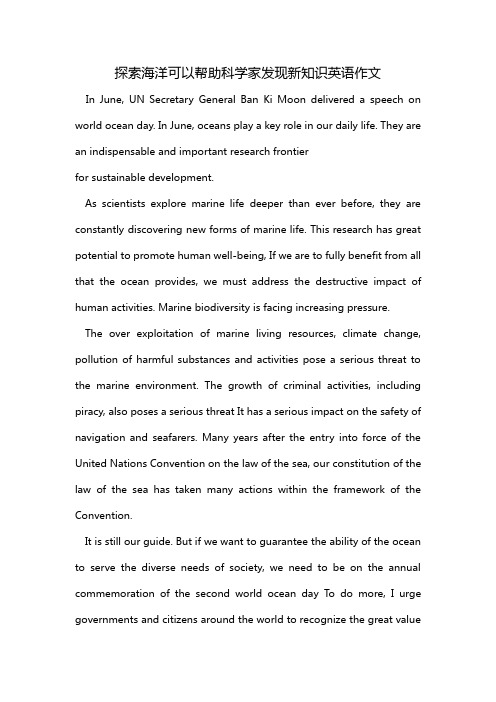
探索海洋可以帮助科学家发现新知识英语作文In June, UN Secretary General Ban Ki Moon delivered a speech on world ocean day. In June, oceans play a key role in our daily life. They are an indispensable and important research frontierfor sustainable development.As scientists explore marine life deeper than ever before, they are constantly discovering new forms of marine life. This research has great potential to promote human well-being, If we are to fully benefit from all that the ocean provides, we must address the destructive impact of human activities. Marine biodiversity is facing increasing pressure.The over exploitation of marine living resources, climate change, pollution of harmful substances and activities pose a serious threat to the marine environment. The growth of criminal activities, including piracy, also poses a serious threat It has a serious impact on the safety of navigation and seafarers. Many years after the entry into force of the United Nations Convention on the law of the sea, our constitution of the law of the sea has taken many actions within the framework of the Convention.It is still our guide. But if we want to guarantee the ability of the ocean to serve the diverse needs of society, we need to be on the annual commemoration of the second world ocean day To do more, I urge governments and citizens around the world to recognize the great valueof the world's oceans and do what they can to ensure that their health and vitality never fail.。
微波辅助合成缺陷型氧化钨及其光催化杀菌机理氧空位的作用
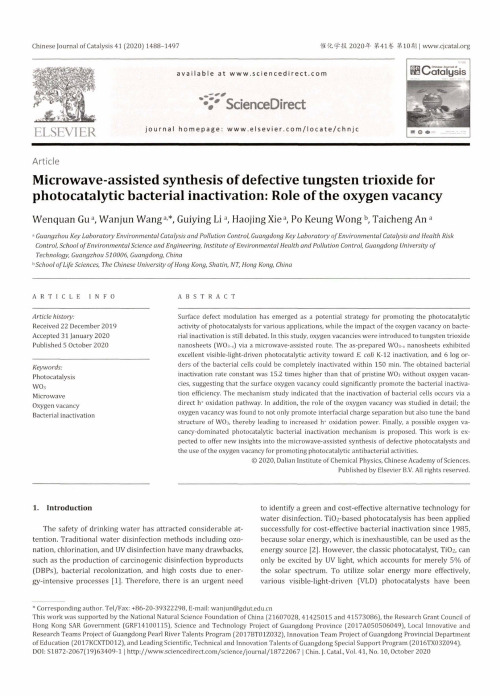
C h inese jo u rn a l o f C ataly sis 41 (2020) 1488-1497催化学报2020年第41卷第10期| w w w.cjcatal.o rgELSEVIERa v a i l ab l e at w w w.sc i e n c ed i re c t.c o mScienceDirectj o u r n a l h o m e p a g e : w w w.e l s e v i e r.c o m /l o c a t e /c h n j cArticleMicrowave-assisted synthesis of defective tungsten trioxide forphotocatalytic bacterial inactivation: Role of the oxygen vacancyWenquan Gua ; Wanjun Wanga >*; Guiying Li a ; Haojing Xiea ; Po Keung Wong b ; Taicheng Anaa Guangzhou Key Laboratory Environmental Catalysis and Pollution Control, Guangdong Key Laboratory o f E nvironmental Catalysis and Health RiskControl, School o f E nvironmental Science and Engineering, Institute o f E nvironmental Health and Pollution Control, Guangdong University of Technology, Guangzhou 510006, Guangdong, Chinab School of L ife Sciences, The Chinese University o f Hong Kong, Shatin, NT, Hong Kong, ChinaA R T I C L EI N F O A B S T R A C TArticle history :Received 22 December 2019 Accepted 31 January 2020 Published 5 October 2020Keywords:Photocatalysis W 03Microwave Oxygen vacancy Bacterial inactivationSurface defect modulation has emerged as a potential strategy for promoting the photocatalytic activity of photocatalysts for various applications, while the impact of the oxygen vacancy on bacterial inactivation is still debated. In this study, oxygen vacancies were introduced to tungsten trioxide nanosheets (W 〇3-x) via a microwave-assisted route. The as-prepared W 〇3-x nanosheets exhibited excellent visible-light-driven photocatalytic activity toward E. coli K-12 inactivation, and 6 log orders of the bacterial cells could be completely inactivated within 150 min. The obtained bacterial inactivation rate constant was 15.2 times higher than that of pristine W 〇3 without oxygen vacancies, suggesting that the surface oxygen vacancy could significantly promote the bacterial inactivation efficiency. The mechanism study indicated that the inactivation of bacterial cells occurs via a direct h+ oxidation pathway. In addition, the role of the oxygen vacancy was studied in detail; the oxygen vacancy was found to not only promote interfacial charge separation but also tune the band structure of WO3, thereby leading to increased h+ oxidation power. Finally, a possible oxygen vacancy-dominated photocatalytic bacterial inactivation mechanism is proposed. This work is expected to offer new insights into the microwave-assisted synthesis of defective photocatalysts and the use of the oxygen vacancy for promoting photocatalytic antibacterial activities.© 2020, Dalian Institute of Chemical Physics, Chinese Academy of Sciences.Published by Elsevier B.V. A ll rights reserved.1. IntroductionT h e s a f e t y o f d r i n k i n g w a t e r h a s a t t r a c t e d c o n s i d e r a b l e a t t e n t i o n . T r a d i t i o n a l w a t e r d i s i n f e c t i o n m e t h o d s i n c l u d i n g o z o n a t i o n , c h l o r i n a t i o n , a n d U V d i s i n f e c t i o n h a v e m a n y d r a w b a c k s , s u c h a s t h e p r o d u c t i o n o f c a r c i n o g e n i c d i s i n f e c t i o n b y p r o d u c t s (D B P s ), b a c t e r i a l r e c o l o n i z a t i o n , a n d h i g h c o s t s d u e t o e n e r g y -i n t e n s i v e p r o c e s s e s [1]. T h e r e f o r e , t h e r e i s a n u r g e n t n e e dt o i d e n t i f y a g r e e n a n d c o s t -e f f e c t i v e a l t e r n a t i v e t e c h n o l o g y f o r w a t e r d i s i n f e c t i o n . T i 〇2-b a s e d p h o t o c a t a l y s i s h a s b e e n a p p l i e d s u c c e s s f u l l y f o r c o s t -e f f e c t i v e b a c t e r i a l i n a c t i v a t i o n s i n c e 1985, b e c a u s e s o l a r e n e r g y , w h i c h i s i n e x h a u s t i b l e , c a n b e u s e d a s t h e e n e r g y s o u r c e [2]. H o w e v e r , t h e c l a s s i c p h o t o c a t a l y s t , T i 〇2, c a n o n l y b e e x c i t e d b y U V l i g h t , w h i c h a c c o u n t s f o r m e r e l y 5% o f t h e s o l a r s p e c t r u m . T o u t i l i z e s o l a r e n e r g y m o r e e f f e c t i v e l y , v a r i o u s v i s i b l e -l i g h t -d r i v e n (V L D ) p h o t o c a t a l y s t s h a v e b e e n*Correspondingauthor.Tel/Fax:+86-20-39322298,E-mail:***************.cnThis work was supported by the National Natural Science Foundation of China (21607028, 41425015 and 41573086), the Research Grant Council of Hong Kong SAR Government (GRF14100115), Science and Technology Project of Guangdong Province (2017A 050506049), Local Innovative and Research Teams Project of Guangdong Pearl River Talents Program (2017BT01Z032), Innovation Team Project of Guangdong Provincial Department of Education (2017KCXTD012), and Leading Scientific, Technical and Innovation Talents of Guangdong Special Support Program (2016TX03Z094). DOI: S 1872-2067(19)63409-l | /science/journal/18722067 | Chin. J. Catal., Vol. 41, No.10, October 2020W enquan Gu et al. / Chinese Journal o f C atalysis 41 (2020) 1488-14971489developed for organic pollutant degradation as well as bacterial inactivation, such as BiV〇4 [3] and g-C3N4 [4]_ However, the bacterial inactivation efficiency is still far from satisfactory; consequently, commercial applications are not feasible yet. More powerful strategies are highly desired to improve the photocatalytic bacterial inactivation efficiency.Surface defects engineering has been portrayed as a promising way to promote the photocatalytic activity. Among all the defects studied in semiconductors, oxygen vacancies are one of the most widely distributed in various metal oxides [5]. It has been revealed that oxygen vacancies can simultaneously improve the absorption of photons and the separation of photo-induced charge carriers [6]. In addition, oxygen vacancies on semiconductor surfaces w ere reported to facilitate the dissociative adsorption of reactant molecules, thereby reducing their activation energy and accelerating the reactions for a variety of applications, including H2evolution, organic pollutant degradation, and CO2reduction [7-10]. However, studies on the influence of oxygen vacancies on the photocatalytic bacterial inac-tivation efficiency are rather limited. Therefore, developing oxygen vacancy deficient photocatalysts and studying their effects on the bacterial inactivation efficiency is attractive.Among many semiconductors, WO3is an excellent semiconductor with visible light response, and it has been extensively utilized as a photocatalyst in pollutant degradation [11], water oxidation [12], and gas sensors [13]. Nevertheless, the band gap energy of bulk WO3is relatively large (about 2.7 eV), corresponding to a cutoff wavelength of 460nm; consequently, the solar spectrum in its entirety cannot be utilized effectively [14] . In addition, due to the rapid recombination rate of photo-generated charge carriers and the narrow photon absorption range, pristine W O3exhibits a low photocatalytic activity [15] . Therefore, a number of strategies have been utilized to modify W O3and improve its photocatalytic activity, such as morphology control [16], ion doping [17], heterojunctions formation [18], and surface defects engineering [19]. Recent studies have also revealed that strongly localized surface plasmon resonances (LSPR) w ere detected on defective W〇3-x, which performs a similar function to that of plasmonic noble metals (e.g., Au and Ag) [20,21]. Although few studies have investigated the use of W〇3-based materials for photocatalytic disinfection [22], the influence of the surface oxygen vacancy on the bacterial inactivation efficiency is still a blank field. Conversely, oxygen vacancy-deficient W〇3-x nanosheets are often synthesized by hydrogen thermal treatm ent methods, which suffer from high tem perature owing to the use of explosive hydrogen gas [23], or by hydrothermal method with long reaction time (> 24 h) [24]. Therefore, safe and facile methods are required from the prospect of large-scale application.In this work, a fast microwave-assisted route was successfully used to fabricate WO3-.V. The photocatalytic bacterial inactivation efficiency against E. coli was studied under VL irradia-tion. Considering the as-prepared W〇3-x as an example, the effect of the oxygen vacancy on the bacterial inactivation efficiency was investigated in detail. In addition, the bacterial inactivation mechanism was elucidated in terms of major reactive species and charge separation efficiency. As a proof-of-concept,this work not only provides a simple synthesis method for fabricating photocatalysts with surface oxygen vacancies but also sheds light on the mechanisms for the oxygen vacancy-mediated enhancement of photocatalytic bacterial inactivation efficiency.2. Experimental2.1. Synthesis o f WCh-x nanosheetsAll the reagents used were of analytical grade and were used as received; all the aqueous solutions w ere prepared using deionized water. W〇3-^was prepared by a microwave solvothermal method. Briefly, 1.0 g of WCU (Aladdin Industrial Corporation, 99%), as the W6+ precursor, was dissolved in 200 mL of absolute ethanol with stirring for 35 min to form a transparent yellow solution. Subsequently, the resulting transparent yellow solution was immediately transferred to a Teflon-lined autoclave. After sealing, the autoclave was heated at 150 °C for 4 h in a microwave hydrothermal synthesizer (XH-800G, Beijing Xiang Hu, China). The vessel was cooled naturally to room temperature, and the resulting product was collected by centrifugation. After washing with ethanol and distilled water severally, the final WO3-X product was dried in a vacuum oven at 50 °C overnight. For comparison, commercial W O3(Shanghai Macklin Biochemical Co. Ltd., 99.8%) was used as pristine WO3 without oxygen vacancies.2.2. C ha ra c te riza tio n o f m a te ria lsThe crystal structures of the as-prepared materials were characterized by powder X-ray diffraction (XRD) (Cu K〇, D/max-Ultima IV). The morphologies and microstructures w ere studied by scanning electron microscopy (SEM) (SU8220, Hitachi, Japan) and transmission electron microscopy (TEM) (FE-HRTEM, Talos F200s, FE1 Company). UV-Vis absorption spectra were recorded using a Cary 100 spectrophotom eter (Agilent, USA). X-ray photoelectron spectroscopy (XPS) was performed on an Escalab 250Xi instrument (Thermo Fisher Scientific). All binding energies were calibrated using a carbon contaminant (C Is = 284.8 eV). Electron param eter resonance (EPR) spectroscopy measurements were conducted using a Bruker EMXPluse EPR spectrom eter. The photoluminescence (PL) spectra of the samples w ere obtained using a fluorescence spectrom eter (Edinburgh FLS1000) with a Xe lamp as the excitation source. EIS tests were conducted with a three-electrode cell using an electrochemical workstation (CHI 650E, Shanghai Chenhua Instruments, China). Fluorine-doped tin oxide (FTO) glasses w ere used to prepare the working electrodes. Briefly, 5 mg of WO3-X was mixed with ethanol (0.2 mL) by ultrasoni-cation to produce a slurry. Subsequently, the slurry was inject-ed uniformly onto the FTO glass and dried at 600C. The effective working area was 1 cm2. A saturated Ag/AgCl electrode and a Pt plate were used as the reference electrode and counter electrode, respectively. EIS examinations w ere conducted across the frequency range from/max = 100 kHz to/min = 1 Hz at 0.5 V with a perturbation signal of 5 mV.1490W enquan Gu et al. / Chinese Journal o f C atalysis 41 (2020) 1488-1497(A )L一^ k After reaction No. 71-2450111.203040 5020 (degree)60^70~80400 500 600 700Wavelength (nm)2.3. P ho to c atalytic b a c te ria l in activatio nE. coli was used as the representative bacterium to test thephotocatalytic bacterial inactivation activity, as it is the most common indicator for microbial contamination in drinking water. A 300 W Xe lamp with a UV cutoff filter (A < 420 nm) was used as the light source, and the light intensity was fixed at 194 m W /cm 2. The final bacterial cell density and catalyst concentration w ere adjusted to l x l 〇6 cfu/mL and 500 mg/L, respectively. The detailed experimental procedures regarding bacterial incubation, sampling, and detection of viable cells can be found in our previous report [3]. For comparison, dark control experiments w ere conducted with only W 〇3-x nanosheets, and light control experiments w ere carried out in the absence of WO 3-A nanosheets under light irradiation. The bacterial inactivation experiments w ere conducted in triplicates.3.Results and discussion3.1.C h a rac terizatio n s o f p h otocatalystThe typical XRD patterns of the as-prepared WO 3-X nanosheets are shown in Fig. 1(A). It is observed that the XRD diffraction pattern can be well indexed as monoclinic W 18O 49 (WO 2.72) (JCPDS No. 71-2450, space group: P 2m ). The major peaks located at 23°, 26°, 35°, and 47° can be attributed to (010], (-104), (113), and (020) crystal planes, respectively, which is consistent with previous studies [24,26]. In addition, no other impurities are detected, suggesting that the WO 3-X nanosheet can be successfully synthesized by the microwave solvothermal method at 150 °C for 4 h. The synthesis condition is much milder than that of the conventional solvothermal method (180 °C for 24 h) [24] because microwave heating has been reported to drastically enhance the crystallization kinetics, up to two orders of magnitude, due to the intense super heating localized to the aqueous solution [25]. The starting material was WC16, which is also much cheaper than W(CO)6 used in previous reports [26,27]. Therefore, the present microwave-assisted method in the study shows promise for potential low-cost and large-scale applications. To evaluate the photostability, the WO 3-X nanosheets, after the photocatalytic disinfection reaction, were also tested. The result showed that the crystal structure of W 〇3-x remained unchanged, suggestingthat the as-prepared W 〇3-x nanosheets possess substantial photostability.The optical absorption was evaluated using the UV-Vis absorption spectra. As shown in Fig. 1(B), the pristine WO 3 shows an absorption edge at about 489.5 nm; thus, the band gap energy can be estimated to be 2.53 eV. In contrast, the as-prepared WO 3-X nanosheets exhibit an absorption edge at about 474.5 nm, which corresponds to a band gap energy of 2.61 eV. A blue shift of the band gap for the W 〇3-x nanosheets can be clearly observed, which can be attributed to the quantum confinement effect of the nanomaterials [28-30]. Impressively, it is noted that the WO 3-X nanosheets show a very large absorption tail, which almost covers the entire visible light region (i.e., 500-800 nm). This can be attributed to the surface oxygen vacancies formed on the photocatalysts, which lead to a broad and strong SPR absorption in the visible light region, according to the references [31,32]. As shown in the inset of Fig. 1(B), the colors of the WO 3 samples change from yellowish-green to grayish-blue, suggesting that the light absorption property was enhanced after the introduction of the oxygen vacancies on the WO 3 sample [33].The morphologies of W 〇3-x and pristine W O 3 were studied by SEM and TEM. As shown in Fig. 2(A), the pristine W O 3 particles exhibit large square morphologies with wide size distributions (20-100 |im). Interestingly, the W O 3 nanosheet prepared by the microwave solvothermal method exhibits a morphology with a thickness of < 20 nm and side length of several hundreds of nanometers (Fig. 2(B )). The corresponding EDX spectrum verifies that the sample is constituted by 0 and W elements without other impurities (Fig. 2(C)). The molar ratio of W to 0 in the WO3-X nanosheets is 1:1.73, according to the quantitative analysis of the EDX spectrum, which further suggests that the obtained W O 3 is nonstoichiometric with the oxygen vacancy. The TEM image in Fig. 2(D) further confirms the morphology of the nanosheet with width and length of about 150 nm and 300 nm, respectively. In addition, clear lattice fringes are observed in the HRTEM image (inset of Fig. 2(D)), indicating the high degree of crystallinity of the as-prepared WO3-X. The inter- planar distance is determined to be 0.37 nm, which can be assigned to the (100) crystal plane of the cubic phase of WO3. The N 2 adsorption/desorption of V V O 3-X is determined to be the type-IV isotherm with a hysteresis loop (Fig. SI), which is typical for mesoporous materials. The BET surface areas of W O 3<(•n .e s)X I I S U a l u lF i g . 1. [A] X-Ray diffraction (XRD) patterns of W 03_x nanosheets; (B) UV-Vis DRS spectra of the W 03_x nanosheets and pristine WO 3.W enquan Gu e ta l./C h in ese Journal o f C atalysis 41 (2020) 1488-14971491535534533532531530529528527Binding Energy (eV)535534533532531530529528527Binding Energy (eV)Fig. 3. (A) XPS survey scan of the W 〇3-x nanosheets and pristine WO 3; High-resolution W 4/XPS profiles of th e 〔B) W 〇3-x nanosheets and (C) pristineWO 3; High-resolution 0 Is XPS profiles of the (D) W 〇3-x nanosheets and 〔D〕pristine WO 3.600 400 200Binding Energy (eV)40 3938 37 36 35 34 33Binding Energy (eV)40 39 38 37 36 35 34 33Binding Energy (ev)(C) 心(B)〜2468101214E n e r g y (K e V )Fig. 2_ SEM images of the (A) W 〇3-x nanosheets and 〔B) pristine W O 3; (C 〕ED X spectrum obtained from W 〇3-x ; (D〕TEM and H RTEM images ofW〇3-x.and W 〇3-x were determined to be 7.52 and 30.23 m2/g, respectively. Noticeably, the W 〇3-x nanosheets have much larger specific surface areas than those of the pristine WO 3 nanosheets. In addition, the morphology of the WO 3-X nanosheet favors intimate contact with the cell surfaces by providing more active sites.To clarify the elemental composition and valance states of the W 〇3-x nanosheets, the sample was characterized by XPS. As shown in Fig. 3(A), the co-existence of only W and 0 elements is observed in the survey scan of the WO 3-X nanosheet sample, suggesting the high purity of the WO 3-X nanosheets. The quantitative analysis based on the XPS profiles suggests that the atomic ratio of W :0 is 1:2.78, which is different from the aboveEDX results (1:1.73). Since EDX is insensitive to the quantitative detection of light elements (e.g., C, O, and N) [34,35], the atomic ratio from XPS should be more reliable. The high-resolution XPS profile of the W 4/o rb it shows two intense peaks corresponding to W 4/5/2 and W 4/7/2〔Fig. 3(B )). The peaks can be further deconvoluted into two Gaussian components with peak binding energies of 38.0 and 35.8 eV, which can be well assigned to W 4/5/2 and W 4/7/2 for W 6+, respectively [12,36-38]. The lower binding energy components centered at 37.6 and 35.4 eV represent W 5+ [36,38]. The spin-orbit splitting energy of W 4/5/2 and W 4/7/2 is determined to be 2.2 eV, in agreement with the literature value [32,40]. The obtained atomic ratio of W6+ to W 5+ is 0.55:0.45. In contrast, the peak intensity of W 5+ in pristine WO 3 decreases dramatically, and theatomic ratio of W6+ to W5+ is 0.83:0.17, suggesting that limited oxygen vacancies exist in pristine WO 3 (Fig. 3(C)). The existence of W 5+ on the surface of the WO 3-X nanosheets confirms that the oxygen vacancies are formed on the photocatalyst surface [32]. Fig. 3[D) shows the high-resolution 0 Is spectrum of WO 3-X , which can be deconvoluted into three Gaussian curves centered at 530.4, 531.1, and 532.2 eV. The intense peak at 530.4 eV can be indexed to the lattice oxygen atoms in metal oxides [36,38,40]. The second peak located at 531.1 eV is related to the oxygen species adsorbed in the form of O-C on the catalyst surface, and the third peak at 532.2 eV can be assigned to the surface-adsorbed H 2O [39]. In contrast, the peak intensity corresponding to the adsorbed oxygen is very weak for pristine W 〇3, suggesting the presence of a small number of surface vacancies (Fig. 3(E)). It should be noted that many surface hydroxyl groups are formed on WO 3-X , which favors the trapping of photo-generated e -h+ pairs, thus inhibiting the undesired charge recombination [40].To confirm, once more, the existence of oxygen vacancies,(•n.B)/Qnsusul(•n.e Q )^f s u ii =(•n .e )x .t :s u ii u l{•I r t o /O I S U a l ul1492W enquan Gu et al. / Chinese Journal o f C atalysis 41 (2020) 1488-1497M agnetic Field (G )〇〇Fig. 4. EPR spectra of the as-prepared W 〇3-x nanosheets and pristineW03.EPR was used to detect the oxygen vacancies in the W 〇3-x nanosheets. The EPR spectroscopy technique has been widely used to study paramagnetic vacancies [41]. As shown in Fig. 4, the W 〇3-x displays a sharp signal at a g-value of 2.002, which is reported to come from the electrons trapped at the oxygen vacancies, according to Ref. [42]. In contrast, no such signal is observed in the pristine W 〇3. This confirms that oxygen vacancy defects are successfully generated in the W 〇3-xby the microwave-assisted method, which is in agreement with the above characterization results. Notably, there is a small peak located around 2700 G . This may be due to some unknown W-based defects [43,44], which has also been reported in a previous study [45].3.2.P h o to c atalytic b a c te ria l in activatio n efficiency an dm echanism sThe visible-light-driven photocatalytic inactivation was conducted using E. coli K-12 as the representative microorganism due to the wide distribution [46]. As shown in Fig. 5(A), in the light and dark control experiments, the variable cells remained unchanged after 3 h, indicating that the bacterial cells cannot be inactivated under VL alone and that W 〇3-a has no toxic effect on the E. coli K-12 cells under dark condition. Interestingly, the as-prepared W 〇3-x nanosheets exhibited rem arkable photocatalytic inactivation activities under VL irradiation,as in all, 6 log orders of bacterial cells were completely inactivated within 2.5 h of irradiation. Notably, the bacterial inactivation rate was very low during the first 1 h of irradiation. This is because, at the initial stage, the bacterial defense system produces high amounts of antioxidant enzymes, including CAT, SOD, and Co A , to overcom e the oxidative stress, thereby resulting in reduced inactivation efficiency. Once the defense system is eliminated by more oxidative species with prolonged reaction time, the inactivation efficiency will be dramatically increased. This phenomenon has also been reported in previous studies [3,47,48]. In comparison, only about 1.0 log reduction of variable cells was obtained after 3 h of VL irradiation, with the pristine W O 3 as photocatalysts. To quantitatively understand the E. coli inactivation rate, the inactivation kinetics were investigated using the Geeraerd model [49]. The inactivation kinetics with W O 3 and WO 3-X as the photocatalysts can be fitted into the log-linear-shoulder model (Eq. (1)) using the software, GInaFit:rg ^m a x ^1log(N )=丨叩 1(10丨。
biotechnologicalpotentialofmarinemicrobes
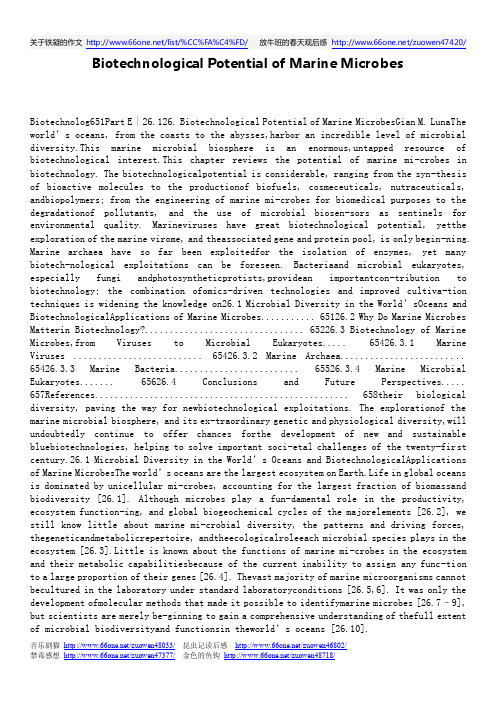
Biotechnological Potential of Marine MicrobesBiotechnolog651Part E | 26.126. Biotechnological Potential of Marine MicrobesGian M. LunaThe world’s oceans, from the coasts to the abysses,harbor an incredible level of microbial diversity.This marine microbial biosphere is an enormous,untapped resource of biotechnological interest.This chapter reviews the potential of marine mi-crobes in biotechnology. The biotechnologicalpotential is considerable, ranging from the syn-thesis of bioactive molecules to the productionof biofuels, cosmeceuticals, nutraceuticals, andbiopolymers; from the engineering of marine mi-crobes for biomedical purposes to the degradationof pollutants, and the use of microbial biosen-sors as sentinels for environmental quality. Marineviruses have great biotechnological potential, yetthe exploration of the marine virome, and theassociated gene and protein pool, is only begin-ning. Marine archaea have so far been exploitedfor the isolation of enzymes, yet many biotech-nological exploitations can be foreseen. Bacteriaand microbial eukaryotes, especially fungi andphotosyntheticprotists,providean importantcon-tribution to biotechnology; the combination ofomics-driven technologies and improved cultiva-tion techniques is widening the knowledge on26.1 Microbial Diversity in the World’sOceans and BiotechnologicalApplications of Marine Microbes........... 65126.2 Why Do Marine Microbes Matterin Biotechnology?................................ 65226.3 Biotechnology of Marine Microbes,from Viruses to Microbial Eukaryotes..... 65426.3.1 Marine Viruses .......................... 65426.3.2 Marine Archaea......................... 65426.3.3 Marine Bacteria......................... 65526.3.4 Marine Microbial Eukaryotes....... 65626.4 Conclusions and Future Perspectives..... 657References................................................... 658their biological diversity, paving the way for newbiotechnological exploitations. The explorationof the marine microbial biosphere, and its ex-traordinary genetic and physiological diversity,will undoubtedly continue to offer chances forthe development of new and sustainable bluebiotechnologies, helping to solve important soci-etal challenges of the twenty-first century.26.1 Microbial Diversity in the World’s Oceans and BiotechnologicalApplications of Marine MicrobesThe world’s oceans are the largest ecosystem on Earth.Life in global oceans is dominated by unicellular mi-crobes, accounting for the largest fraction of biomassand biodiversity [26.1]. Although microbes play a fun-damental role in the productivity, ecosystem function-ing, and global biogeochemical cycles of the majorelements [26.2], we still know little about marine mi-crobial diversity, the patterns and driving forces, thegeneticandmetabolicrepertoire, andtheecologicalroleeach microbial species plays in the ecosystem [26.3].Little is known about the functions of marine mi-crobes in the ecosystem and their metabolic capabilitiesbecause of the current inability to assign any func-tion to a large proportion of their genes [26.4]. Thevast majority of marine microorganisms cannot becultured in the laboratory under standard laboratoryconditions [26.5,6]. It was only the development ofmolecular methods that made it possible to identifymarine microbes [26.7–9], but scientists are merely be-ginning to gain a comprehensive understanding of thefull extent of microbial biodiversityand functionsin theworld’s oceans [26.10].Part E | 26.2652 Part E Marine Microbiology and BiotechnologyIn the United Nations Conventionon Biological Di-versity, biotechnology was defined as [26.11]:any technological application that uses biologicalsystems, living organisms, or derivatives thereof, tomake or modify products or processes for specificuse.There is,however, noagreed upondefinitionof biotech-nology, and definitions may range from the uses ofbiology for the benefit of man to the use of biology tomake money [26.12]. Biotechnology is nowadays expe-riencinganincreasingimportanceattheplanetaryscale,and is expected to increasingly contribute in addressingimportant socio-economic issues of our societies. Theterm blue biotechnologyis typically utilized to describethe aquatic, either marine or freshwater, applications ofbiotechnology [26.4].Marine organisms can be utilized as biotechno-logical agents to provide products and services thathave useful applications for humans and their well-being. Among these, there are new medical technolo-gies, food and feed ingredients (e.g., nutraceuticals),molecules useful in many industrial sectors, and bio-fuels [26.13]. Marine biotechnology has the potentialto contribute significantly to key societal challenges,such as the sustainable supply of healthy and high-quality food, the production of bioenergy (such asbiofuel production from many microalgae), the discov-ery and exploitation of pharmaceuticals or diagnostictools for improving human health, the isolation ofbiopolymers useful in the cosmetic, food and healthindustries, and the synthesis of enzymes, proteins, orbiomaterials of interest in several industrial processes.Marine bioresources (organisms and molecules) can behelpful in increasing stock production in aquacultureprocesses, in controlling infectious diseases of farmedorganisms, as well as in addressing key environmentalissues such as marine pollution (e.g., by the devel-opment of biosensing technologies for environmentalmonitoring, the use of microbes for degradation ofpollutants, or the identification of natural antifoulingtechnologies).This chapter reviews the potential of marine mi-crobes in biotechnology. Marine microbes include rep-resentatives from three domains of life: archaea, bac-teria and eukarya [26.14]. Bacteria and archaea arethe most abundant cells in all known marine ecosys-tems. Microbial eukaryotes, among which unicellularmicrobes (protists) and fungi (including either unicel-lular or multicellular forms), are ecologically importantmembers of marine microbiota, and many studies aredocumenting their once-unimaginable diversity [26.15,16]. This reviewalso coversthebiotechnologicalpoten-tial of marine viruses. Viruses do not have an organizedcell structure, but represent the most abundant bio-logical entities in the oceans. Phages are believed tobe the most diverse biotic component in marine sys-tems, and they are potentially interesting resources forbiotechnology.26.2 Why Do Marine Microbes Matter in Biotechnology?In the twentieth century, research on marine naturalproducts mainly involved the collection of macroor-ganisms from the sea, their extraction, and the anal-ysis of the extracts [26.17]. Most frequently, algaeand marine invertebrates were investigated. Severalmolecules with biological activity were isolated, andmost of them were typically obtained from corals,sponges, or other invertebrates. The first marine bioac-tive compounds, spongouridine and spongothymidine,were isolated from the sponge Cryptotheca crypta inthe Caribbean [26.17]. In the following decades sci-entists proved that these compounds had anticancerand antiviral activities ([26.18] and references therein).These nucleosides were the basis for the synthesis ofAra-C, the first anticancer agent derived from a ma-rine organism, which is currently used in the treatmentof patients affected by leukemia andlymphoma, andthe antiviral drug Ara-A [26.19]. An overwhelmingnumber of bioactive compounds of marine origin havesubsequently been isolated and described, and morethan 15000 marine products have been described sofar [26.20]. Recent estimates report some 20000 ma-rine high-value-added compounds, including a rangeof proteins, carbohydrates, and lipids [26.4,21]. Thereare many examples in the marine pharmacology litera-ture of antitumor and cytotoxic compounds isolated bymarine animals (tunicates, nudibranchs, sponges, octo-corals, bryozoans), algae, fungi, and bacteria [26.22].Some of these substances have created new and excit-ing means for disrupting tumor specific cell signaling,cell division, energy metabolism, or gene expression,and have the potential to revolutionize cancer treat-Biotechnological Potential of Marine Microbes 26.2 Why Do Marine Microbes Matter in Biotechnology? 653Part E | 26.2Fig. 26.1 Marine microbes are a source of bioactivemolecules and secondary metabolites, many of which offerpotential biotechnological opportunities. Photo courtesy ofMarta Scandali (Ancona, Italy)ment [26.22,23]. Marine organisms can be importantsources of useful molecules for treating infectious dis-eases, such as HIV-AIDS (HIV: human immunodefi-ciency virus; AIDS: Aquired Immuno Deficiency Sny-drom; [26.22] and references therein). However, the ap-plication of many substances from marine macroorgan-isms has been hampered by difficulties regarding theirreproduction and scaling up, or problems of supplyingsufficientamountsofthepuresubstance[26.17]. Effortsin trying to cultivatemarine macroorganisms,suchas inthe case of sponges, have been difficult [26.24], despiterecent advances in the ability to cultivate organisms,cells, or their microbial symbionts [26.25]. Therefore,althougha large number of marine natural products iso-lated from macroorganisms has been described frommarine biota, only a few of them have so far enteredpreclinical or clinical trials [26.17].The marine environment is incredibly heteroge-neous, being characterized by a wide variability in themain physical and chemical variables. Temperature canrange from values close to (or below) 0 ? C to up to370 ? C in hydrothermal vents [26.26]. Salinity is typ-ically more uniform across the ocean, but can varysignificantly in specific areas, such as coastal transi-tional environments and estuaries, or reach nearly satu-rating concentrations in hypersalineenvironments,suchas in the deep-hypersaline anoxic basins in the Mediter-ranean Sea [26.27]. The seabed, historically consideredto be a flat, uniform, and biologically inert territory,where hydrostatic pressure can reach values up to 1100bar, and light is typically absent, is now known to in-clude a wide variety of habitats [26.28]. Despite theseapparently inhospitable conditions for supporting life,the seafloor has been recognized as the largest biomeon Earth, and hosts a significant proportion of globalbiomass and biodiversity.Marine organisms, including microbes, have evolv-ed to occupy a broad range of ecological niches, andtheir adaptation across the evolutionary time scale hasoriginated an enormous array of physiological, genetic,and metabolic diversity. The marine biosphere, and par-ticularly its microbial component,is an immense sourceof biodiversity, of bioactive compounds and secondarymetabolites with potential applications in biotechnol-ogy (Fig. 26.1). Marine microbes have evolved specificadaptations to survive under different environmentalchallenges, resulting in different survival mechanisms,growth strategies, and genetic adaptations [26.29].Some examples have been described recently. The pho-tosyntheticpelagic bacteriumProchlorococcusmarinushas adopted a minimalist approach and has reducedthe size of its genome size, to gain a competitive ad-vantage, given thatconditions within its environmentalniche vary onlya little [26.29,30]. Pelagibacter ubique,a marine ?-proteobacterium, has no transposons, extra-chromosomal elements, pseudogenes, or introns, andits genome, consisting of less than 1400 genes, is thesmallest described for a free-living microbe [26.31].The ability of marine microbes to adapt to changesin environmental conditions and to thrive under ex-tremes conditions, coupled with their high geneticplasticity, have influenced their prowess to producecompoundsand secondary metabolites. The marine mi-crobial biosphere represents an important opportunityfor bioprospecting, but the exploration of the poten-tial of marine microbes in terms of the exploitation ofmolecules is still at an early stage [26.4]. As opposed tomacroorganisms, which need to be collected from thesea for sufficient amounts of the searched metabolite tobe extracted, marine microbes can be cultivated in thePart E | 26.3654 Part E Marine Microbiology and Biotechnologylaboratoryinlarge quantities[26.17,32]. Therefore, mi-crobial blue biotechnology has the advantage of beinga more sustainable activity, by avoiding the collectionof marine organisms from their habitat.26.3 Biotechnology of Marine Microbes, from Virusesto Microbial Eukaryotes26.3.1 Marine VirusesVirusesare ubiquitousbioticcomponentsofpelagicandbenthic ecosystems. They influence lateral gene trans-fer, genetic diversity, and bacterial mortality [26.33,34]. Marine viruses were not studied until 1989, butare now recognized as the most abundant biologi-cal entities in the sea (typically 10 7 viruses ml ?1 ;[26.35]. Phage diversity studies were initially restrictedby the requirement for cultivated hosts, but recentapplications of culture-independent techniques have re-vealed an enormous, and unexpected, viral diversityin marine ecosystems [26.35,36]. Archaeal, bacterial,and eukaryotic viruses are cosmopolitan and abundantthroughoutthe world’s oceans [26.37]. Their host rangecovers all known marine organisms, from prokaryotesto fishes and mammals. Phages are particularly abun-dant in marine sediments, and viral infection representsa substantial source of mortality in deep-sea benthicecosystems [26.38]. Many lines of evidence have sug-gested that phage diversity is virtually immense, butstudies describing marine phage diversity are nowadayslimited [26.39].The genetic pool of marine viruses has enormouspotential for biotechnology, but the use of marineviruses as biotechnological agents is still at an earlystage. One potential application can be in medicine, toprovide new solutions to sanitary problems for humansor other organisms. Potential applications of viruses inbiomedicine, some of them already explored, includethe use of viral vectors (gene transfer, gene therapy andvaccine development) and the use of viruses for pro-tein expression and oncolytic viruses (e.g., virotherapyfor treating tumors). There are currently no studies in-volving marine viruses but, given the magnitude of thediversity of marine phages, potential biotechnologicalexploitations can be expected.Phages were used in early forms of biotechnol-ogy to control bacterial infections [26.33,40]. Phagetherapy was abandoned due to contrasting results andthe discovery of antibiotics. It is, however, currentlythe subject of revived interest because of the emer-gence ofantibiotic-resistanceas either a biomedicalandenvironmental problem. Some authors have exploredthe biotechnological usefulness of marine viruses asagents for curing coral diseases. The rapid emergenceof infectious diseases in tropical stony corals is caus-ing serious damage to coral reef ecosystems [26.41].Efrony et al. [26.42] recently isolated two phages ofknown coral bacterial pathogens (Vibrio coralliilyticusand Thalassomonas loyana) and used them incon-trolled aquarium experiments to explore their utilityin coral phage therapy. The results showed that dis-eases could be successfully controlled by the use ofpathogen-specific phages, suggesting the possible use-fulness of phage therapy. Cohen et al. [26.43] and otherauthors recently confirmed the potential applicationof marine viruses in the treatment of coral diseases.Other potential biotechnological applications of marineviruses are in the control of harmful algal blooms. Onjiet al. [26.44] reported on the isolation of two virus-like agents that are able to suppress the growth ofGymnodinium mikimoto, a red-tide-forming marine di-noflagellate.The exploration of the diversity of the marine vi-rome has just begun. The discovery of novel genesand proteins with unknown functions, with potentialbiotechnological applications, can be expected, and de-serves further in-depth investigations.26.3.2 Marine ArchaeaArchaea are evolutionarily unique prokaryotes, as ge-netically distant from bacteria as they are from eu-carya [26.14]. They were historically assumed to liveonly in extremophilic habitats, but two decades of stud-ies have revealed that they are abundant, widespread,and ecologically important members of the marinebiota [26.45,46]. The discoveryofarchaea was a signif-icant breakthrough in the history of biology, leading tothe replacement of the prokaryote/eukaryotedichotomyby a trinity of domains, the archaea, bacteria and eu-karya [26.47].Marine archaea are an interesting source forbiotechnology, however only few fields of biotech-nological exploitations have so far been successfullyBiotechnological Potential of Marine Microbes 26.3 From Viruses to Microbial Eukaryotes 655Part E | 26.3investigated. One was the isolation of enzymes fromsome hyperthermophilic archaea. The vent polymerasewas isolated from the marine archaeon Thermococcuslitoralis, and is currently marketed as a useful alter-native to the widely used Taq deoxyribonucleic acid(Taq DNA: a thermostable DNA polymerase namedafter the thermophilic bacterium Thermus aquaticus;Pfu DNA: a thermostable DNA polymerase named af-ter the thermophilic bacterium Pyrococcus furiosus)polymerase. The Pfu DNA polymerase, isolated bythe hyperthermophilic archaeon Pyrococcus furiosus,is commercially available and possesses a higher fi-delity compared with other conventional Taq DNApolymerases [26.48]. Research into high-fidelity en-zymes from marine archaea is still ongoing and iscontinuously yielding new findings [26.49].Marine archaea have been also exploited in severalindustrial sectors. A heat and acid stable ?-amylase,Valley Ultra-Thin, discovered from a deep-sea hy-drothermal vent archaeon, has been developed to facili-tate the processing of corn into ethanol [26.17]. Marinearchaea can be important producers of biopolymersandbioplastics, such as PHA (polyhydroxyalkanoate), anda source of new secondary metabolites [26.17]. Re-cent studies have pointed out the importance of aquaticarchaea as useful agents for hydrocarbon biodegrada-tion and decontamination of polluted areas [26.50],indicating a potential, underexploited opportunity forbiotechnological investigations.26.3.3 Marine BacteriaBacteria are typically the most abundant and diversemembers of the microbial biota in pelagic and benthicecosystems. They typically outnumber archaea, despiteexceptionsincertain habitats[26.51,52]andare thekeyplayers in biogeochemical processes and the fluxes ofenergy and matter in the ocean. Many bacterial speciesare distributed across marine ecosystems worldwide.Candidatus Pelagibacter ubique, a marine bacterium,is recognized as being one of the most abundant organ-isms on Earth [26.31].Marine bacteria have been largely exploited forseveral biotechnological applications. Most of the ma-rine bioactive compounds that have been successfullyscreened originate from bacteria[26.4]. Marine bacte-ria, especially actinomycetes, are important producersof antimicrobial secondary metabolites and antibiotics(see [26.32] for a review). Marine actinomycetes pro-duce different types of secondary metabolites, a largefraction of which possess biological activities andhave the potential to be developed as therapeuticagents [26.53]. Many other marine bacteria displaysignificant antibacterial activity [26.54], and they arebelieved as an exciting resource for discovering newclasses of therapeutics within the areas of oncology andinfectious diseases [26.55]. Marine cyanobacteria alsoproduce bioactive and cytotoxic molecules that are use-ful as drugs [26.56,57].Unique biosynthetic enzymes from marine bacte-ria have begun to emerge as powerful biocatalysts inmedicinal chemistry and total synthesis [26.58]. Ma-rine bacteria are important producers of biopolymersand biodegradable plastics [26.59], pigments (includ-ing melanin, which is potentially exploitable for sun-screens, dyes, and coloring), cohesive molecules thatare useful as marine cements, and extracellular sub-stances that are useful as surfactants ([26.22] and ref-erences therein).The surface of many marine macroorganisms suchas, to mention only a few, sponges [26.60], al-gae [26.61], tropical stony corals [26.62,63], cold-water corals [26.64], hydroids [26.65], crabs [26.66,67], and fishes, is a particularly interesting niche tostudy marine bacteria and other microorganisms withrespect to biotechnology. The surface of virtually allmarine macroorganisms hosts abundant and diversecommunities of microbes [26.68]. For instance, the tis-sue of stony corals typically harbors an associated anddiversified microbial community (Fig. 26.2), consist-ing of archaea, bacteria, and eukaryotes [26.69,70].Similarly, marine sponges typically contain diverse andabundant microbial communities, made up of bacte-ria, archaea, microalgae, and fungi, which compriseup to 40% of the sponge volume and contribute sig-nificantly to the host metabolism [26.60]. Microbialassociates are believed to have several functions for thesponge, such as stabilization of the sponge skeleton,nutrient uptake,processingofmetabolicwaste, and sec-ondary metabolite production. An increasing numberof studies is documenting the existence of close as-sociations between prokaryotes and higher eukaryoticorganisms, typically forming mutualistic or symbioticrelationships [26.17]. Chemically-driven interactionsare thought to be important in the establishment of re-lationships between epibiotic microorganisms and theireukaryotic hosts. For instance, soft-bodied marine or-ganisms lack obvious structural defense mechanismsand rely on chemical defense, by production of bioac-tive compounds (either by themselves or the associatedmicroflora), to survive [26.71]. Marine invertebrates area rich source of bioactive metabolites, yet recent stud-Part E | 26.3656 Part E Marine Microbiology and Biotechnologya)b)Fig. 26.2a,b Scanning electron microscopy (SEM) im-ages of ( a ) the surface of thestony coral Merulina ampliataand ( b ) the colonial hydroid Ectopleura crocea. The sur-faces of marine macroorganisms are typically hotspotsof microbial diversity, and host a myriad of prokaryoticand eukaryotic microbes with interesting, underexploredbiotechnological potentialities. Photo in b) courtesy ofDr. Cristina Gioia Di Camillo (Polytechnic University ofMarche, Italy)ies have shown that some bioactive compounds thatwere previously ascribed to the host are produced bythe microbial symbionts [26.72]. Epibiotic microbescan produce a plethora of bioactive compounds,includ-ing antibiotics, antiviral, antitumor, and biopolymers.The investigation of the epibiotic microbial commu-nities clearly deserves further study. Similarly, thestudyof other underexplored niches, such as the in-testines of marine organisms [26.73,74], is expectedto provide important contributions to biotechnologicaldevelopments.Marine bacteria have enormous potential applica-tions as biotechnological agents to remediate pollutionin marine systems. These applications can range fromthe useofhydrocarbon-degradingbacteria for oildegra-dation [26.75–77] to the biosynthesis of surfactant andemulsifier molecules to be used in industrial processes,for environmental remediation or as drugs [26.78].Hydrocarbon contamination is an important environ-mental issue, especially in coastal areas subjected tohigh anthropogenic input. Hydrocarbons, and similarlyother pollutants such as pesticides, toxic metals, or her-bicides, reach the marine environment from a widevariety of anthropogenic sources, including oil spills,urban runoff, shipping, and industrial activities. Hydro-carbons typically accumulate in the sediments, posingserious concerns for both the environment and hu-man health. The success of petroleum bioremediationstrategies relies on the ability to provide the optimalconditions to stimulate metabolism of those bacteriathat are able to degrade hydrocarbons. Autochthonousbacterial communities can be used in the bioremedi-ation of contaminated sediments, by stimulating theirdegradation processes with the addition of adequatesubstrates [26.77].The aquaculture industry can also benefit fromseveral biotechnologicalapplicationsderivingfromma-rine bacteria. They can contribute as probiotics, pro-ducers of functional foods and additives to increasebiomass yields, to fight potentially pathogenic bacte-ria and to manage infectious diseases, or may serve asbiodegraders of aquaculture wastes and organic pollu-tants [26.79]. Exploring the potential of marine bacteriaand their metabolites will help in transforming aquacul-ture into a more sustainable and efficient industry.26.3.4 Marine Microbial EukaryotesProtists (including photosynthetic protists or microal-gae) and fungi are abundant and ecologically relevantmembers of marine microbial biota. A plethora of stud-ies have documented their functional role, and recentstudies are documenting their under-recognized ge-netic diversity and the spatial distribution across theocean [26.80,81].Marine fungi form a taxonomically heterogeneousgroup, including obligate marine fungi (those ableto grow and sporulate exclusively in seawater) andfacultative marine fungi, which have a freshwater orterrestrial origin but can grow and sporulate in ma-rine ecosystems [26.82]. There are currently about800 described species of obligate marine fungi, mostlybelonging to ascomycetes, anamorphs, and a few ba-sidiomycetes [26.82]. Many fungi, such as thraus-tochytrids, play an important ecological and biogeo-chemical role in marine ecosystems, and mediate theBiotechnological Potential of Marine Microbes 26.4 Conclusions and Future Perspectives 657Part E | 26.4Fig. 26.3 SEM image of benthic diatoms (Cocconeisspp.). Marine microalgae can be important targets forbiotechnology, especially for biofuel production. Photocourtesy of Dr. Chiara Pennesi (Polytechnic University ofMarche, Italy)degradation of organic matter by the production of ex-tracellular degradative enzymes [26.83]. Historically,fungi have been believed to be rare in marine envi-ronments, but recent studies, based on molecular andmetagenomics approaches, are revealing an unexpecteddiversity of fungal communities in coastal and deep-sea ecosystems [26.84,85]. Recent authors reportedon the discovery of 36 novel marine lineages of ma-rine fungi [26.85]. Marine fungal biotechnology, orblue mycotechnology, is consequently an exciting andpromising area of investigation [26.86]. The number ofbioactive compounds isolated from marine fungi is in-creasing [26.32]. Marine fungiproduce a plethora ofcompounds,amongwhichare anticancer, antibiotic,an-tiangiogenesis,andantiviral compounds,and moleculeshavingantiproliferative activity [26.87]. Deep-sea fungihave been less described in terms of their abun-dance, diversity, and ecological role, but are potentiallyimportant and productive sources of bioactive prod-ucts. Li et al. [26.88] reported about the isolation ofPhialocephalasp.indeep-sea sediments,which synthe-sizes new sorbicillin trimers with cytotoxic properties.Damare et al. [26.89] isolated barotolerant fungi indeep-sea sediments, and reported that some of themproduce proteases active at low temperatures, withbiotechnological potential for waste digestion, foodprocessing, detergents for washing at cold tempera-tures, and preservation. Marine fungi may also serve asuseful pollutant degraders [26.90].Microalgae have an important biotechnological po-tential. Microalgal biotechnology is currently basedon the production and synthesis of food and feed,additives, cosmetics, pigments (carotenoids), and bio-fuel [26.91]. They are used as additives in productsfor human consumption (to enrich the protein con-tent or the nutritional value in food) or as live feedin the aquaculture industry for a variety of farmedorganisms. Marine microalgae are important produc-ers of bioactive compounds [26.92], which are usefulas pharmaceuticals and cosmetics. An noteworthy lineof biotechnological exploitation of microalgae is theiruse for the production of biofuels [26.93]. Combinedwith their fast growth rate, microalgae are consideredone of the few realistic sources for the production ofbiofuels and as being superior to agricultural crop-derived bioethanol [26.94]. Many microalgae, such asdiatoms and dinoflagellates [26.95] naturally accumu-late large amounts of hydrophobic compounds, whichcan exceed 80% of the algal dry weight. Diatoms areamong the most productive and environmentally flex-ible eukaryotic microalgae on the planet (Fig. 26.3).They are responsible for 20% of global carbon fixa-tion by primary production [26.96,97] and have severalcharacteristics that make them useful for large-scalebiofuel cultivation [26.98]. There has been substantialprogress in the development of algal biofuels in the lastdecade,but morefundamentalresearch isneededtobet-ter understand their physiology and metabolism and todevelop efficient large-scale culture systems to growalgae and produce biofuel [26.99]. The biotechnolog-ical potential of diatoms and other marine microalgaealso cover the production of pharmaceuticals, healthfoods, biomolecules, materials relevant to nanotechnol-ogy, and their use as bioremediators of contaminatedwaters [26.100]. The dinoflagellateKarlodiniumvenefi-cum synthesizes karlotoxins, a group of potent toxinsthat cause membrane permeabilization, having possi-ble opportunities to construct new molecules to fighttumors and other human pathologies [26.101,102].26.4 Conclusions and Future PerspectivesOceans are an enormous, untapped and sustainablesource of biotechnological opportunities, yet are un-explored for the most part. Marine microbial diversityis almost unlimited, and offers a huge potential forbiotechnological exploitations. Marine microbes mayposesolutionstoavarietyofissuesrelevantforhumans,Part E | 26658 Part E Marine Microbiology and Biotechnologyby favoring the discovery of more efficacious drugs,the production of biofuel from sustainable sources,the isolation of enzymes that are useful for the in-dustry, the synthesis of biopolymers and biodegrad-able plastics, the remediation of environmental pollu-tion, and the development of a sustainable aquacul-ture. The combination of improved cultivation tech-niques with new culture-independent, omics-driven ap-proaches (such as metagenomics,。
- 1、下载文档前请自行甄别文档内容的完整性,平台不提供额外的编辑、内容补充、找答案等附加服务。
- 2、"仅部分预览"的文档,不可在线预览部分如存在完整性等问题,可反馈申请退款(可完整预览的文档不适用该条件!)。
- 3、如文档侵犯您的权益,请联系客服反馈,我们会尽快为您处理(人工客服工作时间:9:00-18:30)。
J. Ocean Univ. China(Oceanic and Coastal Sea Research)https:///10.1007/s11802-018-3524-8ISSN 1672-5182, 2018 17 (5): 1135-1141/xbywb/E-mail:xbywb@Exploration of Antifouling Potential of the Brown AlgaeLaminaria ‘Sanhai’LI Xiangmin, LI Fengchao, JIAN Huimin , and SU RongguoKey Laboratory of Marine Chemistry Theory and Technology, Ministry of Education, Ocean University of China,Qingdao 266100, China(Received April 14, 2017; revised October 20, 2017; accepted November 13, 2017)© Ocean University of China, Science Press and Springer-Verlag GmbH Germany 2018Abstract Seaweeds are one of the largest producers of biomass in the marine environment. It has been well known that marine algae, especially brown algae was a rich source of biogenic compounds with antifouling potential that could be ideal alternatives of tributyltin (TBT). In this paper, antifouling potential of the brown algae Laminaria ‘sanhai’ was explored. Firstly, the dried alga was extracted and the antialgal and antilarval activities were investigated. The EC50 and LC50 values of crude extract of Laminaria ‘san-hai’ against diatom (Skeletonema costatum) and barnacle larval(Chthamalus challengeri) were 8.9μg mL−1 and 12.0μg mL−1 respec-tively. Then, guided by bioassay, the bioactive substances were isolated by liquid-liquid extraction. The antialgal and antilarval ac-tivities of isolated fraction were improved with the EC50 value of 7.4μg mL−1 against S. costatum and LC50 value of 9.7μg mL−1 against C. challengeri larvae. Identification by IR, Q-TOFMS and GC-MS of the isolated bioactive substances revealed the abun-dance of fatty acids. These fatty acids, most with 16, 18 or 20 carbon atoms, contained myristic, hexadecanoic, oleic, linolenic, ara-chidonic and eicosapentaenoic acids. The results indicated that both the crude extract and the isolated bioactive substances had high antialgal and antilarval activities with no highlighted cytotoxicity which made the brown algae Laminaria ‘sanhai’ a promising source of the environmentally friendly antifoulants.Key words antifouling; artificial breeding; brown algae; crude extract; fatty acids; IR Q-TOFMS and GC-MS1 IntroductionBiofouling, a natural phenomenon, causes many prob-lems to engineered structures such as ships, offshore rigs and jetties by way of increased use of manpower, fuel, material and dry-docking time (Callow et al., 2002; Chambers et al., 2006). Since the early 1960s, tributyltin (TBT) has been used widely in marine paint formulations, but evidence of harmful effects to many non-target or-ganisms was followed (Evans et al., 1995; Omae, 2003; Yebra et al., 2004). Due to the environmental pressure caused by TBT, the International Maritime Organisation (IMO) banned the use of triorganotin-based paints worldwide (Champ, 2000). In order to develop effective but environmentally friendly natural antifoulants, work is progressing worldwide (Hellio et al., 2002; Sipkema et al., 2005; Raveendran et al., 2008).Among these investigations, marine natural products have been highlighted as promising environmental friendly antifoulants (Kjelleberg and steinberg, 1994; Ye-bra et al., 2004; Raveendran et al., 2008). Many organ-isms including marine seaweeds, sponges, corals, ascid-ians and others are believed to produce rich natural anti- * Corresponding author. E-mail: jianhm@ fouling substances that could get rid of fouling organisms (Kharchenko et al., 2012). Some novel natural products for defense purposes had been isolated and investigated, and they all showed antifouling activities with less toxic and more effective compared to the organotin compounds (Proksch et al., 2002; Qian et al., 2010), which indicated the strong antifouling potential of marine natural products. For example, Seaweeds, one of the most studied marine organisms for the isolation of AF compounds, were found to produce a variety of bioactive compounds with high antifouling activity, including fatty acids, lipopetides, amides, alkaloids, terpenoids, lactones, pyrroles and ster-ols (Bhattarai et al., 2006) .The brown macroalgae, one widespread species of seaweeds, had been paid much attention for its high anti-fouling potential and some antifouling compounds were isolated and identified successfully, such as diterpene alcohols from Dictyota menstrualis (Schmitt et al., 1995, 1998), diterpenes from Canistrocarpus cervicornis (Bi-anco et al., 2009), and fatty acids from Sargassum (Hos-sain et al., 2003; Kornprobst, 2005).In this paper, the antifouling potential of the brown macroalga Laminaria ‘sanhai’ was explored. The Lami-naria ‘sanhai’, belonging to Phaeophyceae, Laminariales, Laminariaceae, Saccharina, was achieved by artificial breeding (Laminaria japonica♀ × Saccharina latissimaLI et al . / J . Ocean Univ . China (Oceanic and Coastal Sea Research) 2018 17: 1135-11411136 ♂) with excellent qualities on the basis of the original varieties such as high-temperature-resistance and high production, and it was widely planted in China and com-mercially available. We investigated the antialgal and antilarval activities of the crude extract and isolated bio-active substances from Laminaria ‘sanhai’, and identified their chemical structures by IR, Q-TOFMS and GC-MS.2 Materials and Methods2.1 Sample Pretreatment The Laminaria ‘sanhai’ was provided by Culture Col-lection of Seaweed of the Ocean University of China. The Laminaria ‘sanhai’ sample was rinsed in sterile water and 5% ethanol in order to remove associated micro flora. Cleaned samples were then surface dried by pressing it briefly between the sheets of filter papers and air dried under shade at room temperature in order to prevent photolysis and thermal degradation of metabolites. The dried seaweeds were ground to powder and packed in polyethylene bags and stored in moisture free place be-fore use. 2.2 Extraction and Purification2.2.1 The crude extractThe dried algae was suspended by stirring in 95% etha-nol (200 g in 300 mL) for 4 h at 4℃, the resultant pellet was re-extracted five times in the same way. The alco-holic extracts were combined and evaporated under vac-uum at low temperature (< 40℃). Distilled water (100 mL) and dichloromethane (400 mL) were then added, shaked well and stratified. The organic phase was collected, left dry in presence of Na 2SO 4 for 24 h, filtered and concen-trated under vacuum at low temperature until the volume reaches 5 mL to 10 mL. Following that, the concentrated extract was transferred to a 50 mL centrifuge tube and dried by blowing N 2 (Hellio et al ., 2001). Then the dried crude extract was weighted, finally the dried crude extract dissolved in 0.5 mL DMSO and diluted with Milli-Q wa-ter to obtain a 50 mg mL −1 stock solution stored in refrig-erator before use.2.2.2 Purification of the crude extractThe crude extract was applied in a C18 solid phase ex-traction (SPE) column developed with methanol andeluted with n-hexane and dichloromethane (100%n-hexane, 5:5, 100% dichloromethane) into three frac-tions. The individual fractions were screened for antialgalactivities. Based on the results of antialgal activities, thefractions that was eluted using n-hexane: dichloro-methane (5:5) and 100% dichloromethane were combined and concentrated under vacuum (< 40℃), and then solu-bilized in 20 mL CH 2Cl 2 and partitioned by NaOH (0.2 mol L −1) three times. Then the aqueous phases were com-bined, neutralized by H 2SO 4 (1.0 mol L −1) and extracted by dichloromethane. The finally organic phases resulting from this neutralization were collected and concentrated under vacuum at low temperature (< 40℃) and then dis-solved in 0.2 mL DMSO and diluted into solution (10 mg mL −1) using Milli-Q water before use. Following each step of purification procedure, the antialgal tests were performed to make sure that the bioactive substances were kept.2.3 BioassaysIn this study, diatom Skeletonema costatum (S. co-statum ) and barnacle larval Chthamalus challengeri (C.challengeri ) were used in antifouling activity tests. Dia-toms were the main components of slime films in marine environments. It attached to and reproduced on all but the most toxic surface (Marsalek et al ., 1979; Baier, 1980; Callow et al ., 1986). Barnacle larval was also a common kind of fouling organism worldwide (Swain et al ., 1998; Brady Jr et al ., 2000). They were often used as test or-ganisms for antifouling activities of antifouling com-pounds. In these experiments, we had made sure that the DMSO volume content (< 0.1%) was safe to the target fouling organism (Okumura et al ., 2001). The filteredfresh sea water (GF/F Filter, 0.45 μm) was used in thewhole experiment.2.3.1 Antialgae activities The marine diatom S. costatum was obtained from the Marine Eco-Pollution Chemistry Laboratory of the Ocean University of China. The S. costatum was cultivated in 250 mL Erlenmeyer flasks containing Guillard’s F/2 me-dium under continuous illumination (5000 lux white fluo-rescent lamps) at 20℃ with a 12 h:12 h light:dark cycle.Culture medium were all autoclaved (120℃, 20 min) andthen inoculated with 20 mL cultivated microalgae in the exponential growth phase in 5 L Erlenmeyer flasks con-taining 3 L media under aseptic conditions. The cultureswere shaken three times daily by hand to allow sufficient gas exchange until the microalgae were incubated to the exponential growth period (3 or 4 d) and reached a den-tistry of 105 cells mL −1, then they were collected at the light cycle time for the test. All the experiments were carried out in triplicate. Tests for bioactivity were performed in 250 mL Erlenmeyer flasks filled with 100 mL S. costatum suspension in ex-ponential growth period. The chlorophyll-a fluorescenceintensities (FI) were determinated by a F4500 fluorometer(Hitachi, Japan) as an indicator of algal biomass (Suggettet al ., 2011). A decrease in chlorophyll-a FI indicatedalgae growth inhibition by the extract. The percentage ofgrowth inhibition was calculated as:% inhibition = (FI C – FI T )/ FI C *100,where FI C was the mean chlorophyll-a fluorescence in-tensity of the controls and FI T was the mean chloro-phyll-a fluorescence intensity of the test samples. 2.3.2Antilarval activities The Chthamalus challengeri were collected from the in-tertidal zone in Zhanqiao, Qingdao. After collected,LI et al . / J . Ocean Univ . China (Oceanic and Coastal Sea Research) 2018 17: 1135-11411137washed the sludge and other organisms away and dried in shade, the Chthamalus challengeri was cultured with fresh filtered seawater. Under light irradiation, the adult barnacle released larvae. The larvae were collected, cul-tured for 24 h, and then could be used in the antilarval experiments.The anti-larval experiments were conducted in sterile 3 mL 24-well polystyrene plates. The filtered seawater (0.45 μm) was used as control. Each well contained about 30 barnacle larvae in 3 mL of the test solution. The plates were incubated at 25℃ for 24 h in plant growth chamber under in the dark. All tests were performed in triplicate. The antilarval activities were determined by counting the dead and live individuals and expressing the result as a proportion of the total number of larvae in the well (the percentage of larval death).2.4 Statistical AnalysisThe data from antialgal and antilarval tests were ana-lyzed by SPSS Version 11.5 software package. The dif-ferences between the experimental treatments and the control samples were determined using one-way ANOV As followed by Dunnett’s test at 95% confidence level. The EC 50s were calculated as the concentration at 50% of the maximal effect of S. costatum compared with the control and the LC 50s as the concentration at which 50% of larvae were dead compared with the control. And they were computed from the test results using probit analysis.2.5 Identification of the Isolated Bioactive SubstancesThe isolated bioactive compounds were analyzed and identified by IR, Bruker maXis quadrupole time-of-flight mass spectrometry (Q-TOFMS) and ITQ-1100 gas chro-matography-mass spectrometry (GC-MS).3 Results and Discussion3.1 Antialgal and Antilarval Activities of the CrudextractFor algal inhibiting test, the proper volumes of the crude extract stock solution were added to algae cultures so that its concentrations were 0, 5.0, 7.5, 10.0, 15.0, and 25.0 μg mL −1, respectively, and the chlorophyll-a FI were recorded every 24 h for 96 h. Based on ANOV A and Dun-nett’s analyses, the crude extract, when tested at 7.5 μg mL −1, displayed significant toxicity when compared to the control (P < 0.05). The crude extract with concentrations below 7.5 μg mL −1 did not inhibit the growth of S. co-statum . But when the crude extract concentration was increased up to 10.0 μg mL −1, the algal growth was sig-nificantly inhibited (Fig.1). The EC 50 of the crude extract against S. costatum was 8.9 μg mL −1.A series of aqueous solutions were prepared for anti-larval activity test by diluting the crude extract stock so-lution with the filtered fresh sea water, and the concentra-tions were set at 0, 5.0, 7.5, 10.0, 20.0 and 30.0 μg mL −1,respectively. After 24 h exposure, the crude extract in-duced C. challengeri larvae death in a dose-dependent manner (Fig.2). Based on ANOV A and Dunnett’s analyses, the crude extract, when tested at 7.5 μg mL −1, displayed significant toxicity when compared to the control (P < 0.05). When the concentration of the crude extract was above 20.0 μg mL −1, more than half of test barnacle larvae were killed and the LC 50 of the crude extract against C. challengeri larvae was 12.0 μg mL −1.Fig.1 Growth curve of S. costatum subjected to different concentrations of the crude extract during a 96 h growthperiod.Fig.2 Lethal effects of the crude extract against C . chal-lengeri larvae after 24 h exposure.Hellio et al . (2002) reported that the dichloromethane extract of Ulva lactuca could inhibit the growth of the diatom Phaeodactylum tricornutum with EC 50 of above 300.0 μg mL −1. They (Hellio et al ., 2001) also found that the crude extract of brown algae Bifurcaria brassicae-formis had antialgal activity and when its concentration was up to 30 μg mL −1, more than 60% attachment of spores of macroalgae (such as Enteromorpha intestinalis ) was inhibited. Águila- Ramírez et al . (2012) presented that the MIC (the minimum inhibitory concentrations) values of crude extracts of Ulva lactuca and Laurencia johnstonii against some species of temperate marine mi-croalgae were ranged between 10 and 25 μg mL −1. The antifouling activities of the dichloromethane extract of Himanthalia elongata and crude extract of Lyngbay ma-LI et al . / J . Ocean Univ . China (Oceanic and Coastal Sea Research) 2018 17: 1135-11411138 juscula were also reported with with an LC 50 of 96.2 μg mL −1 against Amphibalanus amphitrite (Maréchal and Hellio, 2011) and an LC 50 of 20 μg mL −1 against Amphi-balanus amphitrite , respectively (Tan et al ., 2010). Comparatively, the crude extract of Laminaria ‘sanhai’ had significantly higher antialgal and antilarval activities.3.2 Antialgal and Antilarval Activities of thePurified Bioactive SubstancesThe stock solution of purified substances was added toalgae cultures to make its concentrations 0, 2.5, 5.0, 7.5,10.0, and 20.0 μg mL −1, respectively, and the chloro-phyll-a FI were recorded every 24 h for 96 h (Fig.3). Based on ANOV A and Dunnett’s analyses, the purified substances, when tested at 7.5 μg mL −1, displayed signifi-cant toxicity when compared to the control (P < 0.05). The purified substances at concentration below 5.0 μg mL −1 had no obvious algal growth inhibition and when the concentration of the purified substances reached upto10.0 μg mL −1, the FI of S. costatum was too weak to be detected. The EC 50 of purified substances toward S. co-statum was determinated as 7.4 μg mL −1. Aqueous solutions of 0, 5.0, 7.5, 15.0, 25.0 and 30.0 μgmL−1 purified substances were prepared for antilarval activity test. Exposure to the purified substances for 24 hFig.3 Growth curve of S.costatum subjected to different concentrations of purified substances during a 96 h growth period.Fig.4 Lethal effects of the purified substances against C . challengeri larvae after 24 h exposure.also increased the C. challengeri larvae death in a dose- dependent manner (Fig.4). Based on ANOV A and Dun-nett’s analyses, the purified substances, when tested at 7.5 μg mL −1, displayed significant toxicity when compared to the control (P < 0.05). More than half of the larvae were dead at the concentration of 15.0 μg mL −1 compared to the control. And when the concentration of the purified sub-stance reached up to 25.0 μg mL −1, all of the tested larvae died. The LC 50 of purified substances toward C. chal-lengeri larvae was 9.7 μg mL −1. Some researchers had reported some natural bioactive compounds with antifouling potential. The palmitic acids from macroalgae Sargassum muticum could inhibit thegrowth of the diatom Cylindrotheca closterium with an EC 50 value of 45.5 μg mL −1(Bazes et al ., 2009). Two fatty acids, 9, 12-Octadecadienoic acid) and n-Hexadecanoicacid, isolated from Laurencia brandenii , showed antilar-val acitivities with the EC 50 of 0.912 mg mL −1and LD 50 of 1.48 mg mL −1against Balanus amphitrite settlement, re-spectively (Manilal et al ., 2010), the bis-1-oxaquinolizi-dine alkaloids isolated from marine sponge Haliclona exigua showed settlement inhibition activity against bar-nacle cypids with LC 50 of 18.07 μg mL −1(Raveendran et al ., 2008), and the linear diterpenes from brown algae Bifurcaria bifurcate had a LC 50 value of 38.3 μg mL −1against cyrids of Balanus amphitrite (Maréchal et al ., 2004). Recently, Cho reported that the chromanol class isolated from brown algae Sargassum horneri with EC 50 of 0.08-1.79 μg mL −1 against diatom Navicula annexa and EC 50 of 1.4-6.4 μg mL −1 agaist mussel larvae settlement (Cho, 2013). From the above, the purified bioactive sub-stances from Laminaria ‘sanhai’ had high antialgal and antilarval activities and could be considered as a promis-ing source of natural antifoulingcompounds.3.3 Identification of the Bioactive CompoundsThe bioactive substances with high antialgal and anti-larval activities was isolated through the bioassay-guided isolation procedure, in which, the alkali washing and ex-traction under acidic conditions were key steps that indi-cated that the bioactive substances should be fatty acid compounds. After bioassays, the isolated bioactive sub-stances were subjected to IR, Q-TOFMS and GC-MSFig.5 IR analysis of the bioactive compounds.LI et al . / J . Ocean Univ . China (Oceanic and Coastal Sea Research) 2018 17: 1135-11411139analysis for chemical structure elucidation.3.3.1 IR identification of the bioactive compounds The IR analysis result was shown in Fig.5. There were three strong peaks. Two of the peaks’ wave-numbers were about 3000 which was in accord with the IR wave number of the carboxylic acid hydroxyl (3400 2500 cm −1).The wave number of about 1700 was the IR characteristic peak of carbonyl group in carboxylic acid. So the IR de-tection confirmed the existence of carboxyl group and the isolated bioactive substances should be mainly composed of fatty acid compounds.3.3.2 Q-TOFMS identification of the bioactivecompoundsNegative mode ionization that removed a hydrogen ion was developed to screen for possible candidate com-pounds (Fig.6). SmartFormula3D was used to identify the fragment and give the sum formula information in the analysis. The bioactive compounds contained 16, 18 or 20carbon atoms based on the molecular formula (Table 1). So the isolated bioactive compounds should be a series of unsaturated and saturated fatty acids that contains 16 or 18 carbon atoms based on the molecular formula pro-vided by Q-TOFMS and the functional group provided by the IR. The exact chemical structures of the isolated bio-active compounds would be given by the following GC- MS analysis.Table 1 the molecular formulae, average mass accuracies of the observed fragmentsQ-TOFMSEntry[M-H] −Molecular formula1 227.1966 C 14H 27O 22 255.2265 C 16H 31O 23 277.2103 C 18H 29O 24 279.2260 C 18H 31O 25 281.2416 C 18H 33O 2 6 283.2567 C 18H 35O 2 7 301.2100 C 20H 29O 28 303.2255C 20H 31O2Fig.6 Negative mode ionization of the bioactive compounds provided by the Q-TOFMS.3.3.3 GC-MS identification of the bioactive compounds Results from the optimized GC-MS analysis indicated the main active ingredients of the isolated bioactive sub-stances were ten fatty acids, oxalic acid, myristic acid,hexadecanoic acid, octadecanoic acid, 9-octadecenoic acid, 9,12-octadecadienoic acid, 6,9,12-octadecatrienoic acid, 9,12,15-octadecatrienoic acid, 6,9,12,15-Eicosatet-raenoic acid, 5,8,11,14-eicosatetraenoic acid and cis-5, 8, 11, 14, 17-eicosapentaenoic acid (Fig.7), they were a se-ries of unsaturated and saturated 16, 18 or 20 carbon fatty acids. Among those fatty acid compounds, Octadecanoicacid accounts for 21.64% by GC peak area integrationwith total area detected normalized to 100%, hexa-decanoic acid for 20.11%, oxalic acid for 18.49%, oleicacids for 8.86%, and myristic acid for 6.29% (Table 2). Some fatty acids could exert a high level of inhibition against certain species of microorganism (Willett and Morse, 1966; Miller et al ., 1977) and people have attach-ed much importance to fatty acids as a promising alterna-tive to TBT (Russel, 1991; Bhattarai et al ., 2006). Hexa-decanoic acid, isolated from macroalgae Sargassum mu-ticum , showed good antialgal activity against the diatomTable 2 The compounds identified by GC-MS analysisEntryTime (min)Name of the compoundPeak area (%)1 6.487 Myristic acid 6.292 7.801 Hexadecanoic acid 20.113 9.010 Octadecanoic acid 21.644 9.132 9-Octadecenoic acid 8.865 9.396 9,12-Octadecadienoic acid 4.32 6 9.583 6,9,12-Octadecatrienoic acid 1.57 7 9.791 9,12,15-Octadecatrienoic acid 2.808 10.007 6,9,12,15-Eicosatetraenoic acid 4.989 11.182 5,8,11,14-Eicosatetraenoic acid 3.58 10 11.802 cis-5,8,11,14,17-Eicosapentaenoic acid 2.99LI et al . / J . Ocean Univ . China (Oceanic and Coastal Sea Research) 2018 17: 1135-11411140 Cylindrotheca closterium with an EC 50 value of 45.5 μg mL −1 (Bazes et al ., 2009). Octadecanoic acid, 5,8,11,14- Eicosatetraenoic acid, and 5,8,11,14,17-Eicosapen-tae- noic acid, isolated from two congeneric sponges from Hong Kong and the Bahamas, had been proved with highly antibacterial activities (Lee et al ., 2007). Suber-gorgic acid, produced by a gorgonian, inhibited settle-ment of Balanus amphitrite larvae with LC 50 value of 200 μg mL −1 (Qi et al ., 2008). Myristic and hexadecanoic ac-ids, isolated from marine fungus Aureobasidium pullulans , showed high inhibition effects against Skeletonema co-statum and Amphibalanus amphitrite larval with an EC 50 of 49.4 μg mL −1 and LC 50 of 22.2 μgmL −1 respectively (Gao et al ., 2013). Comparatively, the fatty acid com-pounds isolated from Laminaria ‘sanhai’ exerted much higher antifouling activity against S. costatum and C. challengeri .Fig.7 GC-MS analysis of the bioactive compounds.4 ConclusionsIn conclusions, the bioactive substances isolated from artificial breeding Laminaria ‘sanhai’ had high antialgaland antilarval activities, and eleven fatty acid compounds were identified as the main active ingredients . Unlike thehigh toxicity and environmental persistency of the or-ganotin compounds, those natural bioactive compounds are reversible toxicity and non-residual effect, whichmade them promising sources of environmental friendly antifoulants. Acknowledgements This study was supported by the National Natural Sci-ence Foundation of China (No. 41376106), the NationalKey Research and Development Program (No. 2016YFC1402101) and the research program from Na-tional Marine Hazard Mitigation Service (No.2014AA060). We are thankful to Prof. Tao Liu of Col-lege of Marine Life of Ocean University of China for providing the Laminaria ‘sanhai’. References Águila-Ramírez, R. N., Arenas-González, A., Hernández-Guerrero, C. J., González-Acosta, B., Borges-Souza, J. M.,Véron, B., Pope, J., and Hellio, C., 2012. Antimicrobial and antifouling activities achieved by extracts of seaweeds from Gulf of California, Mexico actividades antimicrobianay anti- incrustante obtenidas de los extractos de algas marinas del Golfo de California, México. Hidrobiológica , 22 (1): 8-15. Baier, R. E., 1980. Substrata influences on adhesion of micro-organisms and their resultant new surface properties. In: Ad-sorption of Microorganisms to Surfaces . Bitton, G ., and Mar-shall, G ., eds., Wiley, New York, 59-104.Barbosa, J. P., Fleury, B. G ., da Gama, B. A. P., Teixeira, V . L., and Pereira, R. C., 2007. Natural products as antifoulants in the Brazilian brown alga Dictyota pfaffii (Phaeophyta, Dic-tyotales). Biochemical Systematics and Ecology , 35 (8): 549- 553.Bazes, A., Silkina, A., Douzenel, P., Fa, F., Kervarec, N., Morin, D., Berge, J. P., and Bourgougnon, N., 2009. Investigation of the antifouling constituents from the brown alga Sargassum muticum (Yendo) Fensholt. Journal of Applied Phycology , 21 (4): 395-403.Bhadury, P., and Wright, P. C., 2004. Exploitation of marine algae: Biogenic compounds for potential antifouling applica-tions. Planta , 219 (4): 561-578.Bhattarai, H. D., Lee, Y . K., Cho, K. H., Lee, H. K., and Shin, H. W., 2006. The study of antagonistic interactions among pe-lagic bacteria: A promising way to coin environmental friendly antifouling compounds. Hydrobiologia , 568 (1): 417- 423.Bianco, E. M., Teixeira, V . L., Pereira, R. C., de Souza, A. M., Nucci, P., Afonso, I. F., Rodrigues, C. R., and Castro, H. C., 2009. Brown seaweed defensive chemicals: A structure-ac- tivity relationship approach for the marine environment.Natural Product Communications , 4 (2): 173-178.Brady Jr., R. F., and Singer, I. L., 2000. Mechanical factors fa-voring release from fouling release coatings. Biofouling , 15 (1-3): 73-81.Callow, M. E., 1986. Fouling algae from ‘in-service’ ships. Botanica Marina , 24 (4): 351-358.Callow, M. E., and Callow, J. A., 2002. Marine biofouling: Asticky problem. Biologist , 49 (1): 1-5. Chambers, L. D., Stokes, K. R., Walsh, F. C., and Wood, R. J.,2006. Modern approaches to marine antifouling coatings. Surface and Coatings Technology , 201 (6): 3642-3652. Champ, M. A., 2000. A review of organotin regulatory strategies, pending actions, related costs and benefits. Science of the To-tal Environment , 258 (1): 21-71.Cho, J. Y ., 2013. Antifouling chromanols isolated from brown alga Sargassum horneri . Journal of Applied Phycology , 25 (1):299-309.Culioli, G ., Ortalo-Magné, A., Valls, R., Hellio, C., Clare, A. S.,and Piovetti, L., 2008. Antifouling activity of meroditerpe-noids from the marine brown alga Halidrys siliquosa . Journal of Natural Products , 71 (7): 1121-1126. Evans, S. M., Leksono, T., and McKinnell, P. D., 1995. Tribu-tyltin pollution: Adiminishing problem following legislation limiting the use of TBT-based anti-fouling paints. MarinePollution Bulletin , 30 (1): 14-21.Galbraith, H., and Miller, T. B., 1973. Physiological effects oflong chain fatty acids on bacterial cells and their protoplasts. Journal of Applied Bacteriology , 36 (4): 647-658.Hellio, C., De La Broise, D., Dufosse´, L., Le Gal, Y ., and Bourgougnon, N., 2001a. Inhibition of marine bacteria by ex-tracts of macroalgae: Potential use for environmentallyfriendly antifouling paints. Marine Environmental Research , 52 (3): 231-247.。