Localized States and Resultant Band Bending in Graphene Antidot Superlattices
2022年教育部考试中心考研英语模拟试题(新题型4)
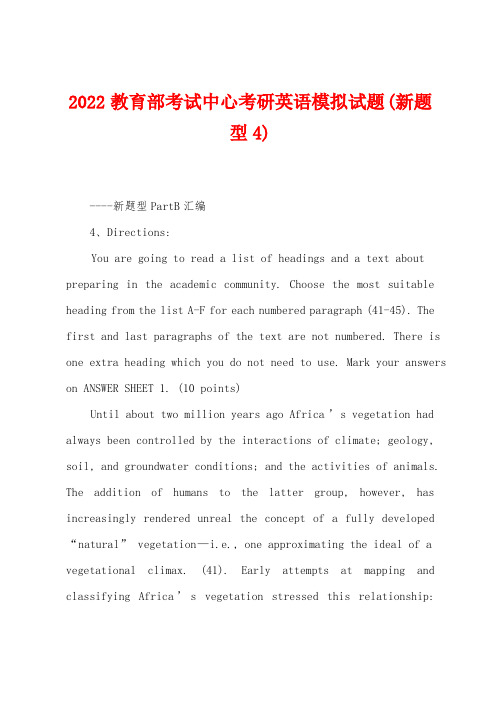
2022教育部考试中心考研英语模拟试题(新题型4)----新题型PartB汇编4、Directions:You are going to read a list of headings and a text about preparing in the academic community.Choose the most suitable heading from the list A-F for each numbered paragraph(41-45).The first and last paragraphs of the text are not numbered. There is one extra heading which you do not need to use. Mark your answers on ANSWER SHEET 1. (10 points)Until about two million years ago Africa’s vegetation had always been controlled by the interactions of climate; geology, soil, and groundwater conditions; and the activities of animals. The addition of humans to the latter group,however,has increasingly rendered unreal the concept of a fully developed “natural” vegetation—i.e., one approximating the ideal of a vegetational climax.(41).Early attempts at mapping and classifying Africa’s vegetation stressed this relationship:sometimes the names of plant zones were derived directly from climates.In this discussion the idea of zones is retained only ina broad descriptive sense.(42). In addition, over time more floral regions of varying shape and size have been recognized.Many schemes have arisen successively,all of which have had to take views on two important aspects: the general scale of treatment to be adopted, and the degree to which human modification is to be comprehended or discounted.(43).Quite the opposite assumption is now frequently advanced. An intimate combination of many species—in complex associations and related to localized soils, slopes, and drainage—has been detailed in many studies of the African tropics. In a few square miles there may be a visible succession from swamp with papyrus, the grass of which the ancient Egyptians made paper and from which the word“paper”originated,through swampy grassland and broad-leaved woodland and grass to a patch of forest on richer hillside soil,and finally to juicy fleshy plants on a nearly naked rock summit.(44). Correspondingly, classifications have differed greatlyin their principles for naming,grouping,and describing formations: some have chosen terms such as forest,woodland,thorn-bush, thicket, and shrub for much of the same broad tracts that others have grouped as wooded savanna (treeless grassy plain) and steppe (grassy plain with few trees).This is best seen in the nomenclature, naming of plants, adopted by two of the most comprehensive and authoritative maps of Africa’s vegetation that have been published: R. W. J. Keay’s Vegetation Map of Africa South of the Tropic of Cancer and its more widely based successor, The Vegetation Map of Africa,compiled by Frank White.In the Keay map the terms“savanna”and“steppe” were adopted as precise definition of formations, based on the herb layer and the coverage of woody vegetation; the White map, however, discarded these two categories as specific classifications.Yet any rapid absence of savanna as in its popular and more general sense is doubtful.(45).However,some100specific types of vegetation identified on the source map have been compressed into14broader classifications.[A] As more has become known of the many thousands of African plant species and their complex ecology, naming, classification,and mapping have also become more particular, stressing what was actually present rather than postulating about climatic potential.[B] In regions of higher rainfall, such as eastern Africa, savanna vegetation is maintained by periodic fires. Consuming dry grass at the end of the rainy season,the fires burn back the forest vegetation, check the invasion of trees and shrubs, and stimulate new grass growth.[C] Once, as with the scientific treatment of African soils, a much greater uniformity was attributed to the vegetation than would have been generally accepted in the same period for treatments of the lands of western Europe or the United States.[D] The vegetational map of Africa and general vegetation groupings used here follow the White map and its extensive annotations.[E] African vegetation zones are closely linked to climatic zones, with the same zones occurring both north and south of the equator in broadly similar patterns.As with climatic zones, differences in the amount and seasonal distribution of precipitation constitute the most important influence on the development of vegetation.[F]Nevertheless,in broad terms,climate remains the dominant control over vegetation.Zonal belts of precipitation,reflection latitude and contrasting exposure to the Atlantic and Indian oceans and their currents,give some reality to related belts of vegetation.[G]The span of human occupation in Africa is believed to exceed that of any other continent. All the resultant activities have tended, on balance, to reduce tree cover and increase grassland; but there has been considerable dispute among scholars concerning the natural versus human-caused development of most African grasslands at the regional level.答案41.F 42.A 43.C 44.G 45.D总体分析本文是一篇介绍非洲植被讨论的科普性文章。
广义联邦滤波器的全局最优性

(2)
T T , H k = [ H1 k , H 2k , · · · ,
T T HN ,V is a zero-mean white k] , V k = V Gaussian noise, whose covariance matrix is Rk , and Rk = diag {R1k , R2k , · · · , RN k }. Thus
With the development of information technology, decentralized filtering[1−3] and federated filtering[4−10] have been widely applied to multisensor information fusion. They have fast computing speed due to their suitable algorithm structure for parallel computing. Fault detection, fault isolation, and system reconfiguration can be achieved with the inherent analytic relation between the input variables and the state variables of the subsystem, so that the reliability of the whole system may be improved. Therefore, they are remarkably superior to the centralized Kalman filtering in computing efficiency and fault tolerance. In proving the optimality of federated filters, Carlson constructed an augmented system, where the variance upper bound technique was used to eliminate the correlation among several local filters. Then, the global optimal estimation was achieved with an uncorrelated fusion algorithm and the information sharing principle. However, in the high-dimensional situation, the computation amount of the above approach will increase sharply. Another approach is the minimum structure. A master filter only contains the common state, and the information sharing is limited to the common state between the master filter and the local filters. Considering the influence of the common state on the bias state, the common state after the information fusion is used to reset the common state of the local filters and also used as the observation feedback to correct the bias state of the local filters. Reference [7] analyzed the above approach theoretically, and pointed out that the minimum structure approach is based on the hypothetical condition that the state estimated error between the local filters and the master filter is uncorrelated. Usually this hypothetical condition is difficult to satisfy and only the suboptimal solution can be obtained. In this paper, based on the decentralized filtering algorithm and the information sharing principle, it is proved that the global filtering of federated filters is optimal only when the dimensions of the master filter and the local filters are totally equal. Meanwhile, the generalized federated filter is structured, where the dimensions of the master filter and the local filters are different, and the feedback correction by which the generalized federated filter may realize the global optimality is presented. The validity of the approach is theoretically proved and its effectiveness is demonstrated by its application to an integrated navigation
异位妊娠双语教学资料
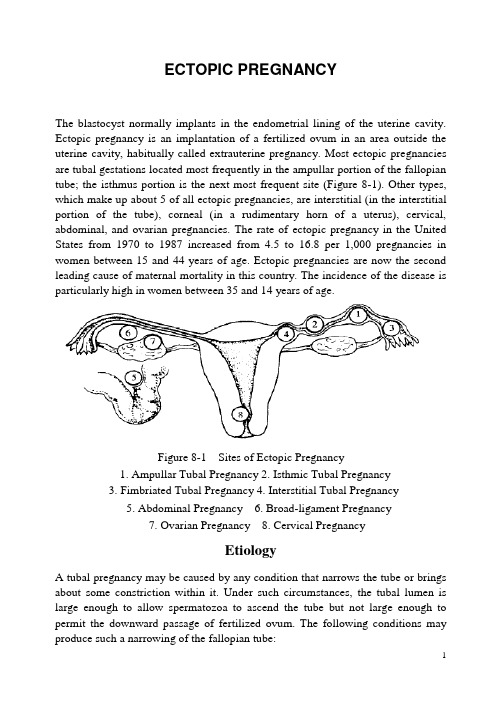
ECTOPIC PREGNANCYThe blastocyst normally implants in the endometrial lining of the uterine cavity. Ectopic pregnancy is an implantation of a fertilized ovum in an area outside the uterine cavity, habitually called extrauterine pregnancy. Most ectopic pregnancies are tubal gestations located most frequently in the ampullar portion of the fallopian tube; the isthmus portion is the next most frequent site (Figure 8-1). Other types, which make up about 5 of all ectopic pregnancies, are interstitial (in the interstitial portion of the tube), corneal (in a rudimentary horn of a uterus), cervical, abdominal, and ovarian pregnancies. The rate of ectopic pregnancy in the United States from 1970 to 1987 increased from 4.5 to 16.8 per 1,000 pregnancies in women between 15 and 44 years of age. Ectopic pregnancies are now the second leading cause of maternal mortality in this country. The incidence of the disease is particularly high in women between 35 and 14 years of age.Figure 8-1 Sites of Ectopic Pregnancy1. Ampullar Tubal Pregnancy2. Isthmic Tubal Pregnancy3. Fimbriated Tubal Pregnancy4. Interstitial Tubal Pregnancy5. Abdominal Pregnancy6. Broad-ligament Pregnancy7. Ovarian Pregnancy 8. Cervical PregnancyEtiologyA tubal pregnancy may be caused by any condition that narrows the tube or brings about some constriction within it. Under such circumstances, the tubal lumen is large enough to allow spermatozoa to ascend the tube but not large enough to permit the downward passage of fertilized ovum. The following conditions may produce such a narrowing of the fallopian tube:1.Previous pelvic inflammatory disease involving the tubal mucosa and producingpartial agglutination of opposing surfaces, for example, Chlamydia, gonorrheal salpingitis.2. Previous inflammatory processes of the external peritoneal surfaces of the tube, for example, puerperal and postabortal infections.3. Endometriosis of the tubal wall and lumen.4. Developmental abnormalities resulting in a segmental narrowing of the tubes or excessive length or kinking.5. Previous abdominal or tubal surgery with resultant scarring and adhesions. Failed tube ligation and a history of previous ectopic pregnancy also increase the risk for an ectopic pregnancy that implants in the fallopian tube. Women who have one ectopic pregnancy have10% to 20% chance that a subsequent pregnancy will also be ectopic. This is because salpingitis that leaves scarring is usually bilateral.6. Previous tubal sterilization.7. Use of contraception that prevents intrauterine pregnancy, such as intrauterine contraceptive devices (IUDs) or low-dose progesterone agents, is associated with increased risk of ectopic pregnancy.PathologyNatural History of Tubal PregnancyTubal Abortion The frequency of tubal abortion depends in part upon the implantation site. Tubal abortion is common in ampullary tubal pregnancy, whereas rupture is the usual outcome with isthmic pregnancy. The immediate consequence of tubal hemorrhage is further disruption of the connection between the placenta and membranes and the tubal wall. If placental separation is complete, all of the products of conception may be extruded through the fimbriated end into the peritoneal cavity. At this point, hemorrhage may cease and symptoms eventually disappear.Some bleeding usually persists as long as products remain in the oviduct. Blood slowly trickles from the tubal fimbria into the peritoneal cavity and typically pools in the recto uterine cul-de-sac. If the fimbriated extremity is occluded, the fallopian tube may gradually become distended by blood, forming a hematosalpinx. (Figure 8-2)After incomplete tubal abortion, pieces of the placenta or membranes may remain attached to the tubal wall and, after becoming surrounded by fibrin,give rise to a placental polyp. The process is similar to that in the uterus after an incomplete abortion.Tubal Rupture The invading, expanding products of conception may rupture the oviduct at any of several sites. Before sophisticated methods to measure chorionic gonadotropin were available, many cases of tubal pregnancy ended during the first trimester by intraperitoneal rupture. As a rule, whenever there is tubal rupture in the first few weeks, the pregnancy is situated in the isthmic portion of the tube. When the fertilized ovum is implanted well within the interstitial portion, rupture usually occurs later. (Figure 8-3)Rupture is usually spontaneous, but it may be caused by trauma associated with coitus or a bimanual examination. With intraperitoneal rupture, the entire conceptus may be extruded from the tube, or if the rent is small, profuse hemorrhage may occur without extrusion. In either event, the woman commonly shows signs of hypovolemia. If an early conceptus is expelled essentially undamaged into the peritoneal cavity, it may reimplant almost anywhere, establish adequate circulation, survive, and grow. This outcome is most unlikely, however, because of damage during the transition. The conceptus, if small, may be resorbed or, if larger may remain in the cul-de-sac for years as an encapsulated mass or even become calcified to form a lithopedion.Abdominal Pregnancy If only the fetus is extruded at the time of rupture, the effect upon the pregnancy will vary depending on the extent of injury sustained by the placenta. The fetus dies if the placenta is damaged appreciably, but if the greater portion the placenta retains its tubal attachment, further development ispossible. The fetus may then survive for some time, giving rise to an abdominal pregnancy. Typically, in such cases, a portion of the placenta remains attached to the tubal wall and the periphery grows beyond the tube and implants on surrounding structures.Broad-ligament Pregnancy When original zygote implantation is toward the mesosalpinx, rupture may occur at the portion of the tube not immediately covered by peritoneum, and the gestational contents may be extruded into a space formed between the folds of the broad ligament. This is designated an intraligamentous or broad-ligament pregnancy.Uterine changesThe uterus undergoes some of the changes associated with early normal pregnancy, including softening of the cervix and isthmus and an increase in size.The degree to which the endometrium is converted to deciduas is variable. The finding of uterine deciduas without trophoblast suggests ectopic pregnancy but is not absolute. In 1954, Arias-Stella described, as had others before him, these changes. Enlarged epithelial cells with nuclei that are hypertrophic, hyperchromatic, lobular, and irregularly shaped. Cytoplasm may be vacuolated and foamy, and occasional mitoses are found. These endometrial changes-the Arias-Stella reaction-are not specific for ectopic and may occur with a normal implantation. External bleeding---seldom severe---is seen commonly in cases of tubal pregnancy and is uterine in origin from degeneration and sloughing of the uterine deciduas. Soon after embryonic death, the decidua degenerates and is usually shed in small pieces. Occasionally it is cast off intact, as a decidual cast of the uterine cavity.Clinical ManifestationClinical Manifestations of a tubal pregnancy are diverse and depend on the site of implantation.In an early ectopic pregnancy, there often are no signs and symptoms. Once the ectopic pregnancy ruptures, however, classic manifestation is present. SymptomsAmenorrhea If implantation occurs in the distal end of the fallopian tube, which can contain the growing embryo longer, the woman may at first exhibit the usual early signs of pregnancy and consider herself to be normally pregnant. About a fourth of women do report amenorrhea; they mistake uterine bleeding that frequently occurs with tubal pregnancy for true menstruation.Abdominal Pain Within 3 to 5 weeks after a missed menstrual period, abdominal pain often develops. The nature, duration, and intensity of pain vary considerably with the length of gestation, site of implantation, and extent of blood loss. Pain isthe predominant symptom of tubal rupture and may be localized on one side or felt over the entire abdomen. The woman may complain of cramping or sharp, sudden, knifelike pain, often of extreme severity. Referred shoulder pain may be present when intraperitoneal bleeding has extended to the diaphragm and irritated the phrenic nerve.Vaginal Bleeding Vaginal bleeding, which occurs when the embryo dies and the deciduas begins to slough, often appears scant and dark brown, and may be intermittent or continuous.Shock and Syncope Depending on the amount of blood loss, the woman may or may not manifest syncope, hypotension, tachycardia, and other symptoms of shock. Hypovolemic shock is a major concern because systemic signs of shock may be rapid and extensive without external bleeding. Women with a ruptured ectopic pregnancy may often present with hypovolemia and shock.Pelvic Mass In some cases, there is gradual disintegration of tubal wall followed by slow leakage of blood into the lumen, peritoneal cavity, or both. Gradually, however, trickling blood collects in the pelvis, more or less walled off by adhesions, and a pelvic hematocele results.SignsGeneral Condition Before rupture, vital signs generally are normal. Blood pressure will fall, pulse rise and shock may present only if bleeding continues and hypovolemia becomes significant. After acute hemorrhage, the temperature may be normal or even low. Temperatures up to 38℃may develop, but higher temperatures are rare in the absence of infectionAbdominal Examination Exquisite tenderness on abdominal is demonstrable in most wo men with ruptured or rupturing tubal pregnancies. Gradually, the woman's abdomen becomes rigid from peritoneal irritation. Abdominal mass may be palpable in some women. With extensive infiltration of blood into the tubal wall, the mass may be firm.Pelvic Examination If blood is slowly seeping into the peritoneal cavity, the umbilicus may develop a bluish tinge (Cullen's sign). The woman may have continuing extensive or dull vaginal and abdominal pain; movement of the cervix on pelvic examination may cause excruciating pain. A tender mass is usually palpable in Douglas' cul-de-sac on vaginal examination. Because of placental hormones, in some cases, the uterus grows during the first3 months of a tubal gestation to nearly the same size as it would with a normal pregnancy. Its consistency may be similar as long as the fetus is alive.Diagnostic TestsTest of Human Chorionic Gonadotropin (hCG)Ectopic pregnancy cannot be diagnosed by a positive pregnancy test alone. The key issue, however, is whether the woman is preg nant. In virtually all cases of ectopic gestation, human chorionic gonadotropin (p-hCG) can be detected in serum, but usually at markedly reduced concentrations compared with normal pregnancy.Ultrasonography Vaginal sonography is more accurate than abdominal sonography in identifying an ectopic pregnancy. With sonographic absence of a uterine pregnancy, a positive pregnancy test, fluid in the cul-de-sac, and an abnormal pelvic mass, ectopic pregnancy is almost certain.Culdocentesis This is a simple technique for identifying hemopentoneum. The cervix is pulled toward the symphysis with a tenaculum, and a long 16-or18-gauge needle is inserted through the posterior fornix into the cul-de-sac. If present, fluid can be aspirated; however, failure to do so is interpreted only as unsatisfactory entry into the cul-de-sac and does not exclude an ectopic pregnancy, either ruptured or unruptured. Fluid-containing fragments of old clots, or bloody fluid that does not clot, are compatible with the diagnosis of hemoperitoneum resulting from an ectopic pregnancy. If the blood subsequently clots, it may have been obtained from an adjacent perforated blood vessel rather than from a bleeding ectopic pregnancy. Laparoscopy Laparoscopy is a definitive diagnosis method in most cases. A characteristic bluish swelling within the tube is the most common finding. It occasionally may be necessary to di agnose rupture of an ectopic pregnancy. Curettage Differentiation between threatened or incomplete abortion and a tubal pregnancy may be accomplished in many instances by office curettage. If an embryo, fetus, or placenta is identified, the diagnosis is apparent. When none of these is identified, tubal pregnancy is a probability and further follow-up is done using serial HCG levels and sonography.ManagementThe therapeutic goal of medical management is early diagnosis of ectopic pregnancy based on a detained health history, physical examination, and selected diagnostic tests. Once the diagnosis is made, surgery is usually necessary. In the past, treatment of an ectopic pregnancy most often was salpingectomy with or without ipsilateral oophorectomy. Medical procedures favoring tubal conservationare now being used more frequently. Earlier diagnosis of ectopic pregnancy through improved techniques has made this type of conservative management possible. If the woman has no history of infertility and no gross evidence of previous salpingitis, a salpingotomy, salpingostomy, or segmental resection and anastomosis may be performed.Postoperative management is directed toward maintaining homeostasis. In cases of ruptured ectopic pregnancy, intervention is aimed at combating shock. Methotrexate, a type of chemotherapy, has been successfully used as an alternative to surgery in some cases. It is a folic acid antagonist that interferes with deoxyribonucleic acid(DNA) synthesis and cell multiplication causing dissolution of the ectopic mass. Methotrexate, 0.4mg/k g·d intramuscularly, 5 days as a therapeutic period. Criteria for its use follow:1. Ectopic sac less than 3cm in diameter2. Tubal pregnancy before tubal abortion or tubal rupture3. No obvious intraperitoneal bleeding4. Blood HCG less than 2,000U/LNursing AssessmentThe initial assessment should focus on the classic triad: amenorrhea followed by abdominal pain and vaginal spotting. Abdominal pain, the most common symptom of ectopic pregnancy is often described as "crampy", "dull", or "restricting to the shoulder and back". The patient also should be questioned about any contraceptive methods, particularly the use of an IUD. A history of previous tubal damage caused by disease or developmental problems further supports the likelihood of a tubal pregnancy.Vital signs are assessed; however, these may not differ markedly from normal values unless tubal rupture and internal bleeding have occurred. During the pelvic examination, the patient is assessed for fullness in the cul-de-sac, cervical pain, and adnexal tenderness. The uterus is generally not enlarged beyond the size of 8 weeks' gestation. Laboratory analysis frequently reveals falling hematocrit and hemoglobin levels and leukocytosis.The amount of bleeding evident may be a poor indicator of the severity of the situation, because blood loss may be concealed in the pelvic cavity. Extensive blood loss leading to hypovolemic shock may be manifested by a rapid, thready pulse; tachypnea; and hypotension. The umbilicus may display a blue tinge (Cullen's sign), indicating bleeding in the peritoneal cavityNursing DiagnosisFluid volume deficit related to the following: bleeding from rupture at implantation site, exc essive fluid loss from surgery.Anticipatory grieving related to loss of pregnancy.Pain related to tubal rupture-peritonitis, intraperitoneal bleeding.Knowledge deficit related to lack of information about treatment and possible complications.Expected Outcomes1. The client will verbalize the pathophysiology of her condition and treatment alternatives.2. The client will demonstrate no signs or symptoms of complications.3. The client will discuss the impact of the loss on her and her family, progressing appropriately through the grieving process.Nursing InterventionsFor the patient with a suspected ectopic pregnancy, the nurse should explain the various diagnostic tests and provide support. When acute rupture of a fallopian tube occurs, the situation presents a surgical emergency requiring nursing care aimed at combating shock. An infusion is maintained so that blood or plasma expanders can be administered as needed to replace losses from the hemorrhage and surgery.Postoperatively, vital signs should be carefully monitored, fluid replacement administered, and intake and output recorded. Oral intake of foods and fluids should be avoided until bowel function has returned to normal. Early ambulation is encouraged. The nurse must accurately record and assess vaginal bleeding and perineal pad count, continuously monitoring the client for signs and symptoms of hemorrhage. The surgical site may require special care and dressings. Patients are often given broad-spectrum antibiotics prophylactically. Steroids are administered to decrease the postoperative inflammation that can contribute to the development of adhesions.Emotional care is directed toward facilitating effective coping by encouraging the patient and her family to verbalize their feelings, allowing them privacy to grieve the death of the fetus, and listening to their concerns about future chances for a successful pregnancy. Information about the causes of ectopic pregnancy may assist them in resolving feelings of guilt and self-blame.Nursing Evaluation1. The client verbalizes the pathophysiology of her condition and treatment alternatives.2. The client demonstrates no signs or symptoms of complications.3. The client discusses the impact of the loss on her and her family, progressing appropriately through the grieving process.。
遥感及其相关术语中英文对照
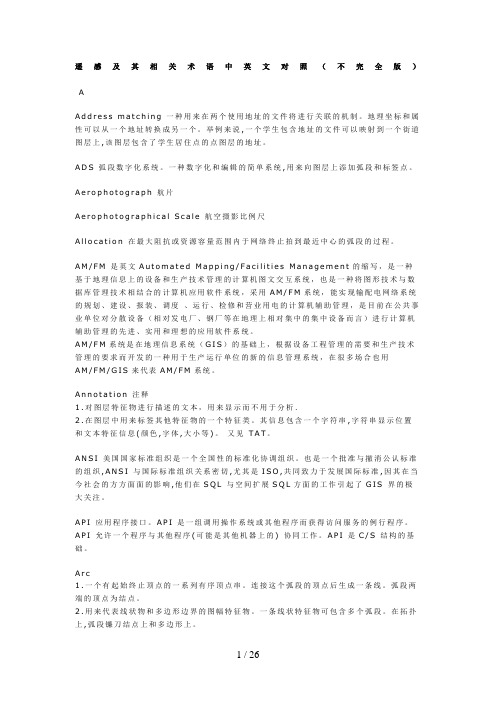
遥感及其相关术语中英文对照(不完全版)AA d d r e s s m a t c h i n g一种用来在两个使用地址的文件将进行关联的机制。
地理坐标和属性可以从一个地址转换成另一个。
举例来说,一个学生包含地址的文件可以映射到一个街道图层上,该图层包含了学生居住点的点图层的地址。
A D S弧段数字化系统。
一种数字化和编辑的简单系统,用来向图层上添加弧段和标签点。
A e r o p h o t o g r a p h航片A e r o p h o t o g r a p h i c a l S c a l e航空摄影比例尺A l l o c a t i o n在最大阻抗或资源容量范围内于网络终止拍到最近中心的弧段的过程。
A M/F M是英文A u t o m a t e d M a p p i n g/F a c i l i t i e s M a n a g e m e n t的缩写,是一种基于地理信息上的设备和生产技术管理的计算机图文交互系统,也是一种将图形技术与数据库管理技术相结合的计算机应用软件系统,采用A M/F M系统,能实现输配电网络系统的规划、建设、报装、调度、运行、检修和营业用电的计算机辅助管理,是目前在公共事业单位对分散设备(相对发电厂、钢厂等在地理上相对集中的集中设备而言)进行计算机辅助管理的先进、实用和理想的应用软件系统。
A M/F M系统是在地理信息系统(G I S)的基础上,根据设备工程管理的需要和生产技术管理的要求而开发的一种用于生产运行单位的新的信息管理系统,在很多场合也用A M/F M/G I S来代表A M/F M系统。
A n n o t a t i o n注释1.对图层特征物进行描述的文本,用来显示而不用于分析.2.在图层中用来标签其他特征物的一个特征类。
其信息包含一个字符串,字符串显示位置和文本特征信息(颜色,字体,大小等)。
又见T A T。
A N S I美国国家标准组织是一个全国性的标准化协调组织。
Hot Spot Tests for Crystalline Silicon Modules
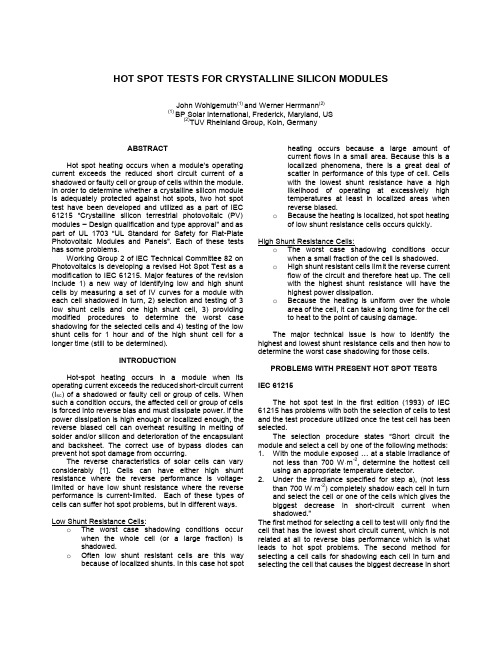
John Wohlgemuth(1) and Werner Herrmann(2) (1) BP Solar International, Frederick, Maryland, US
(2)TUV Rheinland Group, Koln, Germany
Working Group 2 of IEC Technical Committee 82 on Photovoltaics is developing a revised Hot Spot Test as a modification to IEC 61215. Major features of the revision include 1) a new way of identifying low and high shunt cells by measuring a set of IV curves for a module with each cell shadowed in turn, 2) selection and testing of 3 low shunt cells and one high shunt cell, 3) providing modified procedures to determine the worst case shadowing for the selected cells and 4) testing of the low shunt cells for 1 hour and of the high shunt cell for a longer time (still to be determined).
Low Shunt Resistance Cells: o The worst case shadowing conditions occur when the whole cell (or a large fraction) is shadowed. o Often low shunt resistant cells are this way because of localized shunts. In this case hot spot
BoltzTraP. A code for calculation

PROGRAM SUMMARY
Manuscript Title: BoltzTraP. A code for calculating band-structure dependent quantities. Authors: Georg K. H. Madsen, David J. Singh Program Title: BoltzTrap Journal Reference: Catalogue identifier: Licensing provisions: none Programming language: Fortran90 Computer: The program should work on any system with a F90 compiler. The code has been tested with the Intel Fortran compiler. Operating system: Unix/Linux RAM: bytes Up to 2 Gb for low symmetry, small unit cell structures ∗ Corresponding author Email address: georg@chem.au.dk (Georg K. H. Madsen).
R
cRi SR (k) ,
SR (k) =
1 eik·ΛR n {Λ}
(1)
where R is a direct lattice vector, {Λ} are the n point group rotations. The idea of the Fourier expansion is to use more star functions than band energies, but to constrain the fit so ε ˜i are exactly equal to the band-energies, εi and use the additional freedom to minimize a roughness function.(1; 2; 3) The choice of the roughness function, ρR , was discussed by Pickett et al.(3) who found the following expression to be useful for suppressing oscillations between the data-points. ρR =
An introduction to entanglement measures
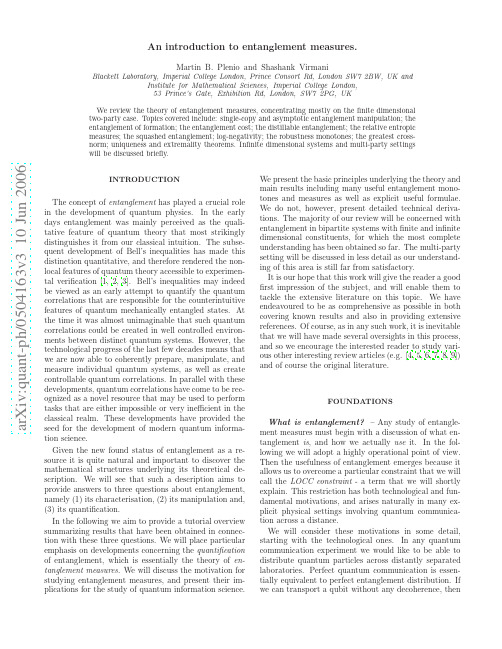
We present the basic principles underlying the theory and main results including many useful entanglement monotones and measures as well as explicit useful formulae. We do not, however, present detailed technical derivations. The majority of our review will be concerned with entanglement in bipartite systems with finite and infinite dimensional constituents, for which the most complete understanding has been obtained so far. The multi-party setting will be discussed in less detail as our understanding of this area is still far from satisfactory. It is our hope that this work will give the reader a good first impression of the subject, and will enable them to tackle the extensive literature on this topic. We have endeavoured to be as comprehensive as possible in both covering known results and also in providing extensive references. Of course, as in any such work, it is inevitable that we will have made several oversights in this process, and so we encourage the interested reader to study various other interesting review articles (e.g. [4, 5, 6, 7, 8, 9]) and of course the original literature.
专业英语(电子与信息工程类)翻译
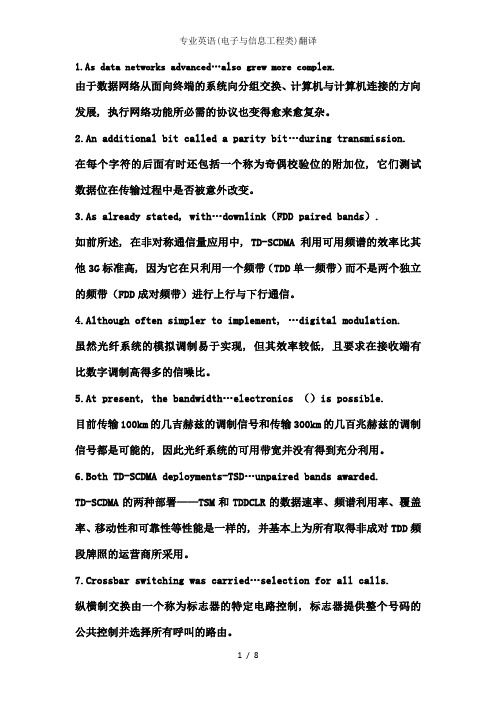
1.As data networks advanced…also grew more complex.由于数据网络从面向终端的系统向分组交换、计算机与计算机连接的方向发展, 执行网络功能所必需的协议也变得愈来愈复杂。
2.An additional bit called a parity bit…during transmission.在每个字符的后面有时还包括一个称为奇偶校验位的附加位, 它们测试数据位在传输过程中是否被意外改变。
3.As already stated, with…downlink(FDD paired bands).如前所述, 在非对称通信量应用中, TD-SCDMA利用可用频谱的效率比其他3G标准高, 因为它在只利用一个频带(TDD单一频带)而不是两个独立的频带(FDD成对频带)进行上行与下行通信。
4.Although often simpler to implement, …digital modulation.虽然光纤系统的模拟调制易于实现, 但其效率较低, 且要求在接收端有比数字调制高得多的信噪比。
5.At present, the bandwidth…electronics ()is possible.目前传输100km的几吉赫兹的调制信号和传输300km的几百兆赫兹的调制信号都是可能的, 因此光纤系统的可用带宽并没有得到充分利用。
6.Both TD-SCDMA deployments-TSD…unpaired bands awarded.TD-SCDMA的两种部署——TSM和TDDCLR的数据速率、频谱利用率、覆盖率、移动性和可靠性等性能是一样的, 并基本上为所有取得非成对TDD频段牌照的运营商所采用。
7.Crossbar sw itching was carried…selection for all calls.纵横制交换由一个称为标志器的特定电路控制, 标志器提供整个号码的公共控制并选择所有呼叫的路由。
Resonance structure in the Li^- photodetachment cross section

arXiv:physics/9703015v1 [physics.atom-ph] 7 Mar 1997
Resonance Structure in the Li− Photodetachment Cross Section
U. Berzinsh,∗ G. Haeffler, D. Hanstorp, A Klinkm¨ uller, E. Lindroth† , U. Ljungblad and D. J. Pegg‡
2
FIGURES
Ion beam
+ + -
Faraday Neutral particle cup detector
+ +
e Glass QD QD plate CEM Laser beam FIG. 1. Portion of the collinear laser ion beam apparatus shБайду номын сангаасwing the interaction and detection regions. Two quadrupole deflectors (Q.D.) are used to merge the two beams.
Typeset using REVTEX 1
Processes such as double excitation and ionization of few-electron atomic systems provide valuable in
G.984.4标准补充修订
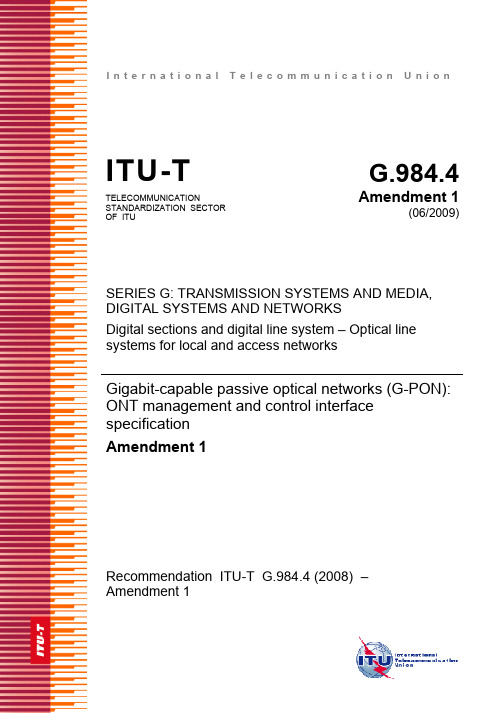
I n t e r n a t i o n a l T e l e c o m m u n i c a t i o n U n i o n ITU-T G.984.4TELECOMMUNICATION STANDARDIZATION SECTOR OF ITU Amendment 1(06/2009)SERIES G: TRANSMISSION SYSTEMS AND MEDIA, DIGITAL SYSTEMS AND NETWORKSDigital sections and digital line system – Optical line systems for local and access networksGigabit-capable passive optical networks (G-PON): ONT management and control interface specificationAmendment 1Recommendation ITU-T G.984.4 (2008) – Amendment 1ITU-T G-SERIES RECOMMENDATIONSTRANSMISSION SYSTEMS AND MEDIA, DIGITAL SYSTEMS AND NETWORKSINTERNATIONAL TELEPHONE CONNECTIONS AND CIRCUITS G.100–G.199GENERAL CHARACTERISTICS COMMON TO ALL ANALOGUE CARRIER-G.200–G.299TRANSMISSION SYSTEMSG.300–G.399INDIVIDUAL CHARACTERISTICS OF INTERNATIONAL CARRIER TELEPHONESYSTEMS ON METALLIC LINESGENERAL CHARACTERISTICS OF INTERNATIONAL CARRIER TELEPHONE SYSTEMSG.400–G.449ON RADIO-RELAY OR SATELLITE LINKS AND INTERCONNECTION WITH METALLICLINESCOORDINATION OF RADIOTELEPHONY AND LINE TELEPHONY G.450–G.499TRANSMISSION MEDIA AND OPTICAL SYSTEMS CHARACTERISTICS G.600–G.699DIGITAL TERMINAL EQUIPMENTS G.700–G.799DIGITAL NETWORKS G.800–G.899DIGITAL SECTIONS AND DIGITAL LINE SYSTEM G.900–G.999General G.900–G.909Parameters for optical fibre cable systems G.910–G.919Digital sections at hierarchical bit rates based on a bit rate of 2048 kbit/s G.920–G.929Digital line transmission systems on cable at non-hierarchical bit rates G.930–G.939Digital line systems provided by FDM transmission bearers G.940–G.949Digital line systems G.950–G.959Digital section and digital transmission systems for customer access to ISDN G.960–G.969Optical fibre submarine cable systems G.970–G.979Optical line systems for local and access networks G.980–G.989Access networks G.990–G.999G.1000–G.1999MULTIMEDIA QUALITY OF SERVICE AND PERFORMANCE – GENERIC AND USER-RELATED ASPECTSTRANSMISSION MEDIA CHARACTERISTICS G.6000–G.6999DATA OVER TRANSPORT – GENERIC ASPECTS G.7000–G.7999PACKET OVER TRANSPORT ASPECTS G.8000–G.8999 ACCESS NETWORKS G.9000–G.9999For further details, please refer to the list of ITU-T Recommendations.Recommendation ITU-T G.984.4Gigabit-capable passive optical networks (G-PON): ONT managementand control interface specificationAmendment 1SummaryAmendment 1 to Recommendation ITU-T G.984.4 contains various updates to ITU-T G.984.4 (2008). A number of editorial corrections and clarifications are included, along with the following substantive changes and extensions to G-PON OMCI.• OMCI for reach extenders• PM extensions for Ethernet bridge ports and circuit emulation services (pseudowires)• Update of OMCI to align with Recommendation ITU-T G.997.1 (2009)• Revision of the VLAN tagging filter data managed entity• A managed entity to control out-of-band file transfer through OMCI• Extended descriptions and OMCI extensions on traffic management and quality of service • A number of additional minor extensions to OMCISourceAmendment 1 to Recommendation ITU-T G.984.4 (2008) was approved on 6 June 2009 by ITU-T Study Group 15 (2009-2012) under Recommendation ITU-T A.8 procedures.Rec. ITU-T G.984.4 (2008)/Amd.1 (06/2009) iFOREWORDThe International Telecommunication Union (ITU) is the United Nations specialized agency in the field of telecommunications, information and communication technologies (ICTs). The ITU Telecommunication Standardization Sector (ITU-T) is a permanent organ of ITU. ITU-T is responsible for studying technical, operating and tariff questions and issuing Recommendations on them with a view to standardizing telecommunications on a worldwide basis.The World Telecommunication Standardization Assembly (WTSA), which meets every four years, establishes the topics for study by the ITU-T study groups which, in turn, produce Recommendations on these topics.The approval of ITU-T Recommendations is covered by the procedure laid down in WTSA Resolution 1.In some areas of information technology which fall within ITU-T's purview, the necessary standards are prepared on a collaborative basis with ISO and IEC.NOTEIn this Recommendation, the expression "Administration" is used for conciseness to indicate both a telecommunication administration and a recognized operating agency.Compliance with this Recommendation is voluntary. However, the Recommendation may contain certain mandatory provisions (to ensure e.g., interoperability or applicability) and compliance with the Recommendation is achieved when all of these mandatory provisions are met. The words "shall" or some other obligatory language such as "must" and the negative equivalents are used to express requirements. The use of such words does not suggest that compliance with the Recommendation is required of any party.INTELLECTUAL PROPERTY RIGHTSITU draws attention to the possibility that the practice or implementation of this Recommendation may involve the use of a claimed Intellectual Property Right. ITU takes no position concerning the evidence, validity or applicability of claimed Intellectual Property Rights, whether asserted by ITU members or others outside of the Recommendation development process.As of the date of approval of this Recommendation, ITU had received notice of intellectual property, protected by patents, which may be required to implement this Recommendation. However, implementers are cautioned that this may not represent the latest information and are therefore strongly urged to consult the TSB patent database at http://www.itu.int/ITU-T/ipr/.© ITU 2010All rights reserved. No part of this publication may be reproduced, by any means whatsoever, without the prior written permission of ITU.ii Rec. ITU-T G.984.4 (2008)/Amd.1 (06/2009)CONTENTSPage1)Clause 2, References (1)2)Clause 3, Definitions (1)3)Clause 4, Abbreviations (1)4)Clause 8.1, Managed entities (2)5)Clause 8.2, Managed entity relation diagrams (3)6)Clause 8.2.4, xDSL service (6)7)New clause 8.2.10 (7)8)Clause 9.1.1, ONT-G (9)9)Clause 9.1.2, ONT2-G (10)10)Clause 9.1.5, Cardholder (10)11)Clause 9.1.10, Protection data (11)12)Clause 9.2.1, ANI-G (12)13)Clause 9.2.3, GEM port network CTP (12)14)Clause 9.2.4, GEM interworking termination point (14)15)Clause 9.2.6, GEM port performance monitoring history data (16)16)Clause 9.3, Layer 2 data services (17)17)Clause 9.3.10, 802.1p mapper service profile (18)18)Clause 9.3.11, VLAN tagging filter data (19)19)Clause 9.3.12, VLAN tagging operation configuration data (22)20)Clause 9.3.13 , Extended VLAN tagging operation configuration data (22)21)Clause 9.3.27, Multicast operations profile (25)22)New clauses 9.3.30 and 9.3.31 (26)23)Clause 9.7, xDSL services (28)24)Clause 9.7.3, xDSL line configuration profile part 1 (28)25)Clause 9.7.5, xDSL line configuration profile part 3 (30)26)Clause 9.7.6, VDSL2 line configuration extensions (32)27)Clause 9.7.7, xDSL channel configuration profile (34)28)Clause 9.7.12, xDSL line inventory and status data part 1 (35)29)Clause 9.7.16, VDSL2 line inventory and status data part 1 (36)30)Clause 9.7.17, VDSL2 line inventory and status data part 2 (37)31)Clause 9.7.19, xDSL channel downstream status data (37)32)Clause 9.7.20, xDSL channel upstream status data (38)33)Clause 9.7.21, xDSL xTU-C performance monitoring history data (38)34)Clause 9.7 (38)Rec. ITU-T G.984.4 (2008)/Amd.1 (06/2009) iiiPage35)Clause 9.8, TDM services (48)36)Clause 9.8.1, Physical path termination point CES UNI (49)37)Clause 9.8.4, CES physical interface performance monitoring history data (51)38)Clause 9.8 (53)39)Clause 9.11.1, Priority queue-G (56)40)Clause 9.11.3, GEM traffic descriptor (58)41)Clause 9.12 (60)42)New clause 9.14 (62)43)Clause 11.1.6, Message identifier (75)44)Clause I.1.1, MIB data sync increase (76)45)Clause I.1.4, Alarm audit and resynchronization (76)46)Clause I.1.5, Table attributes (76)47)Clause I.1.9, Performance monitoring (76)48)Clause I.2.7, Software image download (77)49)Clause II.2.33, End software download (79)50)Clause II.2.27, Test (79)51)Clause II.2.45, Test result (79)52)Appendix III (81)53)Bibliography (83)iv Rec. ITU-T G.984.4 (2008)/Amd.1 (06/2009)Recommendation ITU-T G.984.4Gigabit-capable passive optical networks (G-PON): ONT managementand control interface specificationAmendment 11) Clause 2, Referencesa) Modify the following reference as shown:[ITU-T G.997.1] Recommendation ITU-T G.997.1 (2009), Physical layer management for digital subscriber line (DSL) transceivers.b) Add the following references:[ITU-T G.704] Recommendation ITU-T G.704 (1998), Synchronous frame structures used at 1544, 6312, 2048, 8448 and 44 736 kbit/s hierarchical levels.[ITU-T G.826] Recommendation ITU-T G.826 (2002), End-to-end error performanceparameters and objectives for international, constant bit-rate digital paths andconnections.[ITU-T G.984.6] Recommendation ITU-T G.984.6 (2008), Gigabit-capable passive opticalnetworks (GPON): Reach extension.2) Clause 3, DefinitionsAdd the following clause:3.5 shaping and policing: A shaper causes a flow of input packets to conform to a given PIR/PBS by controlling the release rate/burst size of output packets. This typically results in queuing delay; packets may be dropped if there is a queue overflow because the input rate or burst size is too great.A policer causes a flow of input packets to conform to a given PIR/PBS by immediately dropping packets that exceed PIR/PBS. This typically results in packet loss; packets may be further marked as drop eligible if they exceed CIR/CBS.3) Clause 4, AbbreviationsAdd the following acronyms in alphabetic order:ACL Access Control ListCBS Committed Block SizeDMT Discrete MultitoneFDL Facility Data LinkLBO Line BuildoutBlockSizePBS PeakPCP Priority Code PointR'/S' Reach extender interface to optical trunk lineRAD Rate Adaptation DownshiftRec. ITU-T G.984.4 (2008)/Amd.1 (06/2009) 1RAU Rate Adaptation UpshiftRE ReachExtenderS'/R' Reach extender interface to optical distribution network SRA Seamless Rate Adaptation4) Clause 8.1, Managed entitiesAdd the following entries in alphabetic order to Table 8-1:Table 8-1 – Managed entities of the OMCIManaged entity Required/OptionalDescription ClauseRE ANI-G CR Used for mid-span PON reach extender ANI 9.14.1 Physical path termination pointRE UNICR Used for mid-span PON reach extender UNI 9.14.2RE upstream amplifier CR Used for mid-span PON reach extender upstreamoptical amplifier9.14.3RE downstream amplifier CR Used for mid-span PON reach extenderdownstream optical amplifier9.14.4RE config portal CR Used for non-OMCI configuration method onmid-span PON reach extenders9.14.5RE common amplifier parameters CR Used for monitoring and maintenance of PONreach extender optical amplifiers9.14.6File transfer controller O Used to control out-of-band file transfers 9.12.13 CES physical interfaceperformance monitoringhistory data 2O Used for PM of DS1, E1 and similar CESs 9.8.12CES physical interfaceperformance monitoringhistory data 3O Used for PM of DS1, E1 and similar CESs 9.8.13Ethernet frame performance monitoring history data upstream O Used for PM of upstream Ethernet flows on abridge port9.3.30Ethernet frame performance monitoring history data downstream O Used for PM of downstream Ethernet flows on abridge port9.3.31VDSL2 line configurationextensions 2O Used to configure additional VDSL2 parameters 9.7.26xDSL impulse noise monitorperformance monitoringhistory dataO Used for impulse noise monitoring PM 9.7.27xDSL line inventory and status data part 5 CR Additional xDSL test parameters for G.992.3,G.992.5 Annex C9.7.28xDSL line inventory and status data part 6 CR Additional xDSL test parameters for G.992.3,G.992.5 Annex C9.7.29xDSL line inventory and status data part 7 CR Additional xDSL test parameters for G.992.3,G.992.5 Annex C9.7.302Rec. ITU-T G.984.4 (2008)/Amd.1 (06/2009)5) Clause 8.2, Managed entity relation diagramsa) Throughout clause 8.2, replace the indicated figures with the following:GEM portnetwork CTPFigure 8.2.2-6 − Illustration of N:M bridge-mappingRec. ITU-T G.984.4 (2008)/Amd.1 (06/2009) 3GEM port network CTPGEM portnetwork CTPFigure 8.2.2-7 − Illustration of 1:MP map-filtering4Rec. ITU-T G.984.4 (2008)/Amd.1 (06/2009)Figure 8.2.2-10 − Illustration of multicast serviceb) Add the following figure at the end of clause 8.2.2:GEM portnetwork CTPFigure 8.2.2-11 − Illustration of downstream broadcast configuration6) Clause 8.2.4, xDSL serviceReplace Figure 8.2.4-1 with the following:7) New clause 8.2.10Add the following new clause at the end of clause 8.2:8.2.10 Mid-span PON reach extendersThe PON reach extender is modelled as an ONT (the management entity) containing cardholders and circuit packs whose functions are to extend the reach of one or more PONs. The PON reach extender's own management ONT is understood to exist as a member of one of the extended PONs.Figure 8.2.10-1 – Mid-span PON reach extender core (repeater)NOTE 1 – In many cases, the RE ANI-G and PPTP RE UNI will be implemented on the same circuit pack. If so, the port mapping package can be used to create the hybrid line card.Figure 8.2.10-2 – Mid-span PON reach extender core (optical amplifier)NOTE 2 – In many cases, the RE upstream amplifier and RE downstream amplifier will be implemented on the same circuit pack. If so, the port mapping package can be used to create the hybrid line card.Figure 8.2.10-3 – Mid-span PON reach extender core (hybrid)Figure 8.2.10-4 – Mid-span PON reach extender core (hybrid)Figure 8.2.10-5 – In-band management for mid-span PON reach extender8) Clause 9.1.1, ONT-Ga) Replace:Traffic management option:This attribute identifies the upstream traffic management function implemented in the ONT. There are two options:0 Priority controlled and flexibly scheduled upstream traffic. The trafficscheduler and priority queue mechanism are used for upstream traffic.1 Rate controlled upstream traffic. The maximum upstream traffic ofeach individual connection is guaranteed.With:Traffic management option:This attribute identifies the upstream traffic management function implemented in the ONT. There are three options:0 Priority controlled and flexibly scheduled upstream traffic. The trafficscheduler and priority queue mechanism are used for upstream traffic.1 Rate controlled upstream traffic. The maximum upstream traffic ofeach individual connection is guaranteed by shaping.2 Priority and rate controlled. The traffic scheduler and priority queuemechanism are used for upstream traffic. The maximum upstream traffic of each individual connection is guaranteed by shaping.b) Add the following new attribute:ONT survival time:This attribute indicates the minimum guaranteed time in milliseconds between the loss of external power and the silence of the ONT. This doesnot include survival time attributable to a backup battery. The value zeroimplies that the actual time is not known. (R) (optional) (1 byte)9) Clause 9.1.2, ONT2-GReplace:OMCC version: This attribute identifies the version of the OMCC protocol being used by the ONT. This allows the OLT to manage a network with ONTs that supportdifferent OMCC versions. Release levels of this Recommendation may besupported with the following code points:0x80 G.984.4 (06/04).NOTE – For historic reasons, this codepoint may also appear in ONTs that supportlater versions of G.984.4.0x81 G.984.4 Amd.1 (06/05)0x82 G.984.4 Amd.2 (03/06)0x83 G.984.4 Amd.3 (12/06)0x84 G.984.4 (02/2008)(R) (mandatory) (1 byte)With:OMCC version:This attribute identifies the version of the OMCC protocol being used by the ONT. This allows the OLT to manage a network with ONTs that supportdifferent OMCC versions. Release levels of this Recommendation may besupported with the following code points:0x80 G.984.4 (06/04).NOTE – For historic reasons, this codepoint may also appear in ONTs that supportlater versions of G.984.4.0x81 G.984.4 Amd.1 (06/05)0x82 G.984.4 Amd.2 (03/06)0x83 G.984.4 Amd.3 (12/06)0x84 G.984.4 (02/08)0x85 G.984.4 (2008) Amd.1 (06/09)(R) (mandatory) (1 byte)10) Clause 9.1.5, CardholderWhere Table 9.1.5-1 presently reads:Table 9.1.5-1 − Circuit pack typesCoding Content Description224..242 ReservedModify it to read:Table 9.1.5-1 – Circuit pack types Coding Content Description 224..238 Reserved239 Mid-span PON reachextender UNI The UNI of a mid-span PON reach extender, 2488 Mbit/s downstream and 1244 Mbit/s upstream240 Mid-span PON reachextender ANI The ANI of a mid-span PON reach extender, 2488 Mbit/s downstream and 1244 Mbit/s upstream241 Mid-span PON reachextender upstream opticalamplifierThe 1310 nm wavelength optical amplifier242 Mid-span PON reachextender downstreamoptical amplifierThe 1490 nm wavelength optical amplifier11) Clause 9.1.10, Protection dataModify the description of this managed entity to read as follows:This managed entity models the capability and parameters of PON protection. An ONT that supports PON protection automatically creates an instance of this managed entity.NOTE 1 – Equipment protection is modelled with the equipment protection profile and cardholder managed entities.NOTE 2 – For ONTs that implement reach extender functions, this ME can be used to describe OMCI protection, reach extender R'/S' protection, or both. For reach extender R'/S' protection, the protection type must be 1:1 without extra traffic, because the switching is done on a link-by-link basis, and the protection link is in cold stand-by mode. The instance that pertains to OMCI protection has ME ID = 0. RelationshipsOne instance of this managed entity is associated with two instances of the ANI-G, RE ANI-G or RE upstream amplifier. One of the ANI managed entities represents the working side; the other represents the protection side.AttributesManaged entity id:This attribute uniquely identifies each instance of this managed entity.This ME is numbered in ascending order from 0. (R) (mandatory)(2 bytes)Working ANI-G pointer:This attribute points to the ANI-G, RE ANI-G or RE upstream amplifier managed entity that represents the working side of PON protection. (R) (mandatory) (2 bytes)Protection ANI-G pointer:This attribute points to the ANI-G, RE ANI-G or RE upstream amplifier managed entity that represents the protection side of PON protection.(R) (mandatory) (2 bytes)(Remainder of description remains unchanged)12) Clause 9.2.1, ANI-G Replace:Piggyback DBA reporting:This attribute indicates the ONT's piggyback DBA reporting format capabilities. [ITU-T G.984.3] defines three possible piggyback reporting modes. For reporting mode 0, the single field is the entire report. For reporting mode 1, the DBA report is two fields long. For reporting mode 2, the DBA report is four fields long. Mode 0 is mandatory for ONTs that utilize the piggyback DBA reporting method; modes 1 and 2 are optional. The following coding indicates the ONT's piggyback DBA reporting mode capabilities:0 Mode 0 only1 Modes 0 and 12 Modes 0 and 23 Modes 0, 1 and 24 Piggyback DBA reporting not supported(R) (mandatory) (1 byte)Whole ONT DBA reporting:This attribute indicates that the ONT supports whole ONT DBA reporting (1) as specified in [ITU-T G.984.3], or that it does not (0). (R) (mandatory) (1 byte)With:Piggyback DBA reporting:This attribute indicates the ONT's piggyback DBA reporting format capabilities. [ITU-T G.984.3] defines two possible piggyback reporting modes. For reporting mode 0, the single field is the entire report. For reporting mode 1, the DBA report is two fields long. Mode 0 is mandatory for ONTs that utilize the piggyback DBA reporting method; mode 1 is optional. The following coding indicates the ONT's piggyback DBA reporting mode capabilities:0 Mode 0 only1 Modes 0 and 12 Deprecated3 Deprecated4 Piggyback DBA reporting not supported(R) (mandatory) (1 byte)Whole ONT DBA reporting:This attribute is deprecated. It should be set to 0 by the ONT and ignored by the OLT. (R) (mandatory) (1 byte)13) Clause 9.2.3, GEM port network CTPa) Replace:Port id value:This attribute is the port ID of the GEM port associated with this CTP.(R, W, Set-by-create) (mandatory) (2 bytes)Port id value:This attribute is the port ID of the GEM port associated with this CTP.NOTE 1 – While nothing forbids the existence of several GEM port networkCTPs with the same port id value, downstream traffic is modelled as beingdelivered to all such GEM port network CTPs. Be aware of potential difficultiesassociated with defining downstream flows and aggregating PM statistics.(R, W, Set-by-create) (mandatory) (2 bytes)b) Replace:Traffic management pointer for upstream:If the traffic management option attribute in the ONT-G ME is 0 (priority controlled), this pointer specifies the priority queue-G ME serving this GEM port network CTP. If the traffic management option attribute is 1 (rate controlled), this attribute redundantly points to the T-CONT serving this GEM port network CTP. (R, W, Set-by-create) (mandatory) (2 bytes)Traffic descriptor profile pointer:This attribute points to the instance of the GEM traffic descriptor managed entity that contains the traffic parameters used for this GEM port network CTP ME. This attribute is used when the traffic management option attribute in the ONT-G ME is 1 (rate controlled). (R, W, Set-by-create) (optional) (2 bytes)See also Appendix III.With:Traffic management pointer for upstream:If the traffic management option attribute in the ONT-G ME is 0 (priority controlled) or 2 (priority and rate controlled), this pointer specifies the priority queue-G ME serving this GEM port network CTP. If the traffic management option attribute is 1 (rate controlled), this attribute redundantly points to the T-CONT serving this GEM port network CTP. (R, W, Set-by-create) (mandatory) (2 bytes)Traffic descriptor profile pointer for upstream:This attribute points to the instance of the GEM traffic descriptor managed entity that contains the upstream traffic parameters used for this GEM port network CTP ME. This attribute is used when the traffic management option attribute in the ONT-G ME is 1 (rate controlled), specifying the PIR/PBS to which the upstream traffic is shaped. This attribute is also used when the traffic management option attribute in the ONT-G ME is 2 (priority and rate controlled), specifying the CIR/CBS/PIR/PBS to which the upstream traffic is policed. (R, W, Set-by-create) (optional) (2 bytes) See also Appendix III.c) Replace:Priority queue pointer for downstream:This attribute points to the instance of the priority queue-G used for this GEM port network CTP in the downstream direction. (R, W, Set-by-create) (mandatory) (2 bytes)Priority queue pointer for downstream:This attribute points to the instance of the priority queue-G used for this GEM port network CTP in the downstream direction. It is the responsibility of the OLT to provision the downstream pointer in a way that is consistent with bridge and mapper connectivity. If the pointer is undefined, downstream queueing is determined by other mechanisms in the ONT. (R, W, Set-by-create) (mandatory) (2 bytes)NOTE 3 – If the GEM port network CTP is associated with more than one UNI (downstream multicast), the downstream priority queue pointer defines a pattern (e.g., queue number 3for a given UNI) to be replicated (i.e., to queue number 3) at the other affected UNIs.d) Add the following additional attribute:Traffic descriptor profile pointer for downstream:This attribute points to the instance of the GEM traffic descriptor managed entity that contains the downstream traffic parameters used for this GEM port network CTP ME. This attribute is used when the traffic management option attribute in the ONT-G ME is 2 (priority and rate controlled), specifying the CIR/CBS/PIR/PBS to which the downstream traffic is policed. (R, W, Set-by-create) (optional) (2 bytes)See also Appendix III.14) Clause 9.2.4, GEM interworking termination pointa) Replace:Interworking option:This attribute identifies the type of non-GEM function that is being interworked. The options are:0 UnstructuredTDM1 MAC bridge LAN2 Reserved for future use3 IP data service4 Video return path5 802.1pmapper(R, W, Set-by-create) (mandatory) (1 byte)Service profile pointer:This attribute points to an instance of a service profile, such as:CES service profile-G if interworking option = 0MAC bridge service profile if interworking option = 1IP router service profile if interworking option = 3Video return path service profile if interworking option = 4802.1p mapper service profile if interworking option = 5(R, W, Set-by-create) (mandatory) (2 bytes)Interworking option:This attribute identifies the type of non-GEM function that is being interworked. The options are:0 UnstructuredTDM1 MAC bridge LAN2 Reserved for future use3 IP data service4 Video return path5 802.1pmapper6 Downstreambroadcast(R, W, Set-by-create) (mandatory) (1 byte)Service profile pointer:This attribute points to an instance of a service profile, such as:CES service profile-G if interworking option = 0MAC bridge service profile if interworking option = 1IP router service profile if interworking option = 3Video return path service profile if interworking option = 4802.1p mapper service profile if interworking option = 5Null pointer if interworking option = 6(R, W, Set-by-create) (mandatory) (2 bytes)b) Replace:GAL profile pointer:This attribute points to an instance of the GAL profile. The relationship between the interworking option and the related GAL profile is:Interworking option GAL profile type0 GAL TDM profile1 GAL Ethernet profile2 Reserved for future use3 GAL Ethernet profile for data service4 GAL Ethernet profile for video returnpath5 GAL Ethernet profile for 802.1pmapper(R, W, Set-by-create) (mandatory) (2 bytes)GAL loopback configuration:This attribute sets the loopback configuration when using GEM mode: 0 Noloopback.1 Loopback of downstream traffic after GAL.The default value of this attribute is 0. (R, W) (mandatory) (1 byte)GAL profile pointer:This attribute points to an instance of the GAL profile. The relationship between the interworking option and the related GAL profile is:Interworking option GAL profile type0 GAL TDM profile1 GAL Ethernet profile2 Reserved for future use3 GAL Ethernet profile for data service4 GAL Ethernet profile for video returnpath5 GAL Ethernet profile for 802.1pmapper6 Nullpointer(R, W, Set-by-create) (mandatory) (2 bytes)GAL loopback configuration:This attribute sets the loopback configuration when using GEM mode:0 Noloopback1 Loopback of downstream traffic after GALThe default value of this attribute is 0. When the interworking option is 6 (downstream broadcast), this attribute is not used. (R, W) (mandatory) (1 byte)15) Clause 9.2.6, GEM port performance monitoring history dataReplace:Lost packets:This attribute counts background GEM frame loss. It does notdistinguish between packets lost because of header bit errors or bufferoverflows; it records only loss of information. (R) (mandatory)(4 bytes)Misinserted packets:This attribute counts GEM frames misrouted to this GEM port. (R)(mandatory) (4 bytes)Received packets:This attribute counts GEM frames that were received correctly at themonitored GEM port. (R) (mandatory) (5 bytes)Received blocks:This attribute counts GEM blocks that were received correctly at themonitored GEM port. (R) (mandatory) (5 bytes)Transmitted blocks:This attribute counts GEM blocks originated by the transmitting endpoint (i.e., backward reporting is assumed). (R) (mandatory) (5 bytes) Impaired blocks:This severely errored data block counter is incremented whenever oneof the following events takes place: the number of misinserted packetsreaches its threshold, the number of bipolar violations reaches itsthreshold, or the number of lost packets reaches its threshold.Threshold values are based on vendor-operator negotiation. (R)(mandatory) (4 bytes)。
Conserving approximation for the three-band Hubbard model flat quasiparticle dispersion
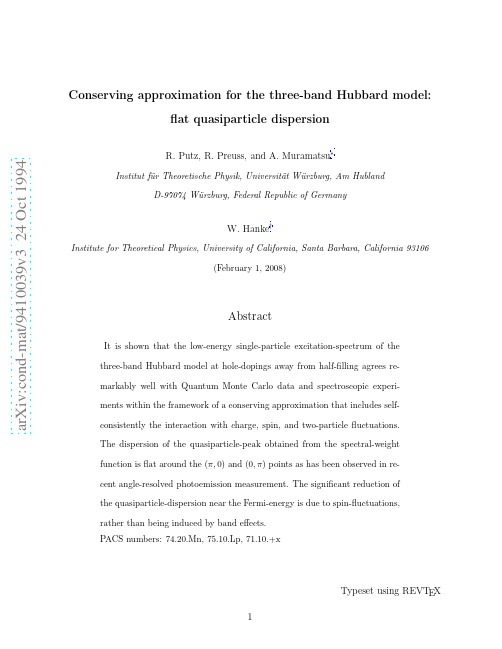
a r X i v :c o n d -m a t /9410039v 3 24 O c t 1994Conserving approximation for the three-band Hubbard model:flat quasiparticle dispersionR.Putz,R.Preuss,and A.Muramatsu ∗Institut f¨u r Theoretische Physik,Universit¨a t W¨u rzburg,Am HublandD-97074W¨u rzburg,Federal Republic of GermanyW.Hanke †Institute for Theoretical Physics,University of California,Santa Barbara,California 93106(February 1,2008)Abstract It is shown that the low-energy single-particle excitation-spectrum of the three-band Hubbard model at hole-dopings away from half-filling agrees re-markably well with Quantum Monte Carlo data and spectroscopic experi-ments within the framework of a conserving approximation that includes self-consistently the interaction with charge,spin,and two-particle fluctuations.The dispersion of the quasiparticle-peak obtained from the spectral-weightfunction is flat around the (π,0)and (0,π)points as has been observed in re-cent angle-resolved photoemission measurement.The significant reduction ofthe quasiparticle-dispersion near the Fermi-energy is due to spin-fluctuations,rather than being induced by band effects.PACS numbers:74.20.Mn,75.10.Lp,71.10.+xTypeset using REVT E XThe nature of quasiparticle excitations in the high-T c superconductors(HTSC)is of central interest in order to characterize the normal-state of these materials.Angle-resolved photoemission spectroscopy(ARPES)[1–4]shows on the one hand,the existence of a Fermi-surface in the normal-state in accordance with Luttinger’s theorem supporting the conven-tional Fermi-liquid concept.On the other hand,these experimentsfind a veryflat dispersion of the quasiparticle excitations near the X=(π,0)and Y=(0,π)points.This extended saddle-point behavior cannot be derived from single-particle calculations(Hartree-Fock,LDA[5]) whichfind,in contrast,a van Hove bandstructure singularity.The importance of many-body correlation effects for such aflat quasiparticle dispersion was revealed in recent Quantum Monte Carlo(QMC)simulations of both the three-band[6]and the one-band Hubbard model[7].Related interpretations were also advanced recently on the basis of QMC sim-ulations of the t-J model[8].However,in spite of the success of the numerical techniques in dealing with strongly correlated systems,the physical interpretation of purely numerical QMC results is often difficult without reference to more transparent theoretical approaches.In this letter one-particle spectra for realistic parameters[6,9,10]of the three-band Hub-bard model are presented based on a conserving approximation[11,12].The obtained spec-tral distribution of the quasiparticle peak agrees remarkably well with QMC-and ARPES results.The main features of the experimental bandstructure,such as theflat dispersion near the Fermi-energy,bandwidth,and Fermi-velocities are reproduced by our theoretical approach.Furthermore,in contrast to the QMC simulations,we are able to distinguish the influence of different many-body scattering channels.We can clearly show that magnetic fluctuations are responsible for the drastic reduction of characteristic energy-scales in the quasiparticle excitation spectrum compared to their mean-field values.Thus,theflatness has a many-body origin,rather than being induced by band effects,a result which may have important implications particularly for mechanisms of superconductivity based on spin fluctuations[13–15].The results presented here were obtained at much lower temperatures than in numerical simulations such that a direct comparison with experiments is possible.Our analysis is based on the two-dimensional three-band Hubbard model[16,17]withthe Hamiltonian H−µN=H0−µN+H U,whereH0−µN= i,σ(εd−µ)n diσ+ j,σ(εp−µ)n pjσ+ <i,j>,σt ij(d†iσp jσ+h.c.),andH U=U d i n di↑n di↓.(1) Here d†iσand p†jσdenote the electron creation operators for Cu-3d x2−y2and O-2p orbitals at sites i and j,respectively with spinσ,and n diσ=d†iσd iσ.The one-electron part H0−µN is determined by the local orbital levelsεd(Cu)andεp(O)with charge-transfer energy ∆=εp−εd,and a Cu-O hopping t ij=±t between nearest-neighbor Cu-O sites<i,j>, where the sign takes into account the phase factors for the2p and3d x2−y2orbitals.µis the chemical potential and the interaction part H U consists of the on-site Coulomb repulsion U d(Cu).The calculations were performed for the parameter set U d=6,∆=4in the hole representation(as everywhere,the energy-unit is t=1).This choice follows from recent QMC studies[6,9,10],where it was shown that the above parameter set leads to a consistent description of several important features of the HTSC.Bickers and Scalapino have developed a propagator-renormalizedfluctuation-exchange (FLEX)approximation[11]for the one-band Hubbard model based on the conserving ap-proximation(CA)of Baym and Kadanoff[18]which has resulted in significantly improved agreement with QMC results[12].Properties of the superconducting state in the same model were also studied recently by that method[19]The starting-point of this self-consistentfield solution is the non-interacting Hamiltonian H0in Eq.1.Diagonalization of H0leads to the eigenvalues E0n(k)(n=1,2,3)which build up the unperturbed bandstructure.The single-particle propagator of the non-interacting system G0can now be expressed in terms of these energies E0n(k)and the corresponding eigenvectors c nν(k):G0(k,ωm) ν,ν′= n c nν(k)c∗nν′(k)frequencies are given byωm=(2m+1)πT,m={...,−2,−1,0,1,2,...}.The Coulomb interaction H U is accounted for within thefield-theoretical FLEX approximation by a power series for the self-energy.This series includes diagrams(symmetric in particle-hole and particle-particle channels)taking into account the interaction of electrons with density(d), spin(s),and particle-particle(p)fluctuations.For the present three-orbital Hubbard model the single-particle self-energy is as follows:Σ(k) dd=TU2dχph(q) 12U2dχph(q) 12−1 .(4)1+U dχpp(q)k stands for the fermionic momenta and Matsubara frequencies(k,ωm),and q for the bosonic (q,νn)ones.χph andχpp are the propagators of non-interacting particle-hole and particle-particlefluctuations:−Tχph(q)=N k G(k+q) dd G(−k) dd.(5) Inserting this self-energy into Dyson’s equation the single-particle propagator has to be computed self-consistently:G(k,ωm) −1= G0(k,ωm) −1− Σ[G,U](k,ωm) ,(6) with G0standing for the single-particle propagator of the non-interacting system.The single-particle excitations are determined by the peaks of the spectral-weight func-tion1A(k,ω)=−is found for the quasiparticle excitation spectra calculated by the FLEX approximation and the spectroscopic measurements.The main features of the experimental data are reproduced by our theoretical approach:Along theΓ—M high symmetry direction wefind a dispersing quasiparticle peak which crosses through the Fermi-energy near k=(π/2,π/2).The two other symmetry lines in Fig.2clearly show the existence of theflat band dispersion near X=(π,0),the most striking result of the ARPES experiments.The quasiparticle peak of A(k,ω)is broadened for excitation energies away fromµdue to strong correlation effects. In fact,it can be seen that not only the position of the maxima in A(k,ω)(dispersion)but also the overall shape of the spectra agrees with the experimental results.The single-particle bandstructure obtained from the low-lying peak in A(k,ω)is depicted in Fig.3in comparison to results of ARPES experiments in Bi2212.In order to discern the influence of different many-body scattering channels,we present the data of three approxi-mative stages:In the Hartree-Fock(HF)or band-theory approximation(dashed line in Fig.3)the HF bandwidth,Fermi-velocities,and the characteristic energy-dispersion at(π,0)are far away from agreement with the experiment.Chargefluctations given by V(d)(q)in Eqs. 3and4tend to reduce these differences(dotted line in Fig.3),but only the inclusion of both longitudinal and transverse magneticfluctuations[22]V(s)(q)leads to aflat quasi-particle band near the Fermi-energy.The particle-particle channel V(p)(q)contributes only in a minor way to the full FLEX calculations(full line in Fig.3)for the given parameter set.Therefore,Fig.3demonstrates that spin-fluctuations are responsible for the reduced energy-scales compared to mean-field calculations,especially for theflat dispersion nearµand the reduced saddle-point energy at(π,0).The most dominant contributions stem from magnetic modes with a wave-vector q near to the antiferromagnetic point(π,π).Finally,Fig.4shows a strong correlation between the energy difference∆≡E(X)−µand the energyωspin of the maximum in Imχspin(q,ω)at the incommensurate wavevector, where Imχspin reaches its largest value in the BZ.This suggests that for dopingsδ<∼0.25, the energy difference∆is locked-in to the magnetic excitations close to the AF-point[23]. Moreover,we observe in our FLEX-calculations a linearω-dependence of ImΣdown toapproximately the same energy-scale.Below this energy-scale,ImΣ∼ω2as expected for a Fermi-liquid.Details of these features will be given elsewhere[24].In summary,we presented the single-particle excitation spectrum of the three-band Hub-bard model within the framework of a conserving approximation.This many-body approach is based on a self-consistent evaluation of Dyson’s equation includingfluctuation-exchange (particle-hole and particle-particle)self-energy contributions.The results are in remarkable agreement with Quantum Monte Carlo data and spectroscopic experiments for low-lying single-particle excitation energies.The low-energy physics of this FLEX calculation is con-trolled by many-bodyfluctuations in the magnetic scattering channel leading to a significant reduction of characteristic bandstructure energy-scales from their mean-field values.We ackowledge helpful discussions with P.Dieterich,N.E.Bickers and,in particular, D.J.Scalapino,who also carefully read the manuscript.The calculations were performed on the Cray Y-MP EL of the Rechenzentrum der Universit¨a t W¨u rzburg and on the Cray Y-MP8of the Leibniz-Rechenzentrum M¨u nchen and of the H¨o chstleistungsrechenzentrum J¨u lich.We thank these institutions for their support.One of us(W.H.)acknowledges the hospitality at the ITP in Santa Barbara and support through the NSF–ITP program. Furthermore,we gratefully acknowledge support by the Deutsche Forschungsgemeinschaft project No.Ha1537/5–2and by the Bavarian High–T c program“FORSUPRA”.REFERENCES∗Permanent address:Institut f¨u r Physik,Universit¨a t Augsburg,Memminger Str.6,D-86135Augsburg,Federal Republic of Germany†Permanent address:Institut f¨u r Theoretische Physik,Universit¨a t W¨u rzburg,Am Hub-land,D-97074W¨u rzburg,Federal Republic of Germany[1]C.G.Olson et al.,Phys.Rev.B42,381(1990).[2]G.Mante et al.Z.Phys.B80,181(1990).[3]D.S.Dessau et al.Phys.Rev.Lett.71,2781(1993).Z.-X.Shen and D.S.Dessau,Phys.Reports,to be published.Care has to be taken for the nomination of the high-symmetry points:The X-point of our two-dimensional cubic lattice corresponds to the¯M-point for the Bi2212compound and the M-point of our calculations corresponds to the X-point in Bi2212.[4]A.A.Abrikosov,J.C.Campuzano and K.Gofron,Physica C214,73(1993).[5]S.Massidda,J.Yu,and A.J.Freeman,Physica C52,251(1988);H.Krakauer and W.E.Pickett,Phys.Rev.Lett.60,1665(1988).[6]G.Dopf,J.Wagner,P.Dieterich,A.Muramatsu,W.Hanke,Phys.Rev.Lett.68,2082(1992).[7]N.Bulut,D.J.Scalapino,and S.R.White,Phys.Rev.Lett.72,705(1994).[8]E.Dagotto,A.Nazarenko,and M.Boninsegni,Phys.Rev.Lett.73,728(1994).[9]G.Dopf,A.Muramatsu,W.Hanke,Phys.Rev.Lett.68,353(1992).[10]G.Dopf,A.Muramatsu,and W.Hanke,Europhys.Lett.17,559(1992).[11]N.E.Bickers and D.J.Scalapino,Annals of Physics193,207(1989).[12]N.E.Bickers and S.R.White,Phys.Rev.B43,8044(1991).[13]D.J.Scalapino,E.Loh,and J.E.Hirsch,Phys.Rev.B34,8190(1986);N.E.Bickers,D.J.Scalapino,and S.R.White,Phys.Rev.Lett.62,961(1989).[14]T.Moriya,Y.Takahashi,and K.Ueda,J.Phys.Soc.Jpn.59,2905(1990).[15]P.Monthoux,A.V.Balatsky,and D.Pines,Phys.Rev.Lett.67,3448(1991);P.Monthoux and D.Pines,Phys.Rev.Lett.69,961(1992).[16]C.M.Varma,et al.,Solid State Comm.62,681(1987).[17]V.J.Emery,Phys.Rev.Lett.58,2794(1987).[18]G.Baym and L.P.Kadanoff,Phys.Rev.124,287(1961);G.Baym,Phys.Rev.127,1391(1962).[19]P.Monthoux and D.J.Scalapino,Phys.Rev.Lett.72,1874(1994).[20]H.J.Vidberg and J.W Serene,J.Low Temp.Phys.29,179(1977).[21]N.Bulut,D.J.Scalapino and S.R.White,Preprint UCSBTH–94–05.[22]D.M.Frenkel and W.Hanke,Phys.Rev.B42,6711(1990).[23]It should be noted that the temperature T=0.01t≈150K is still above the temperaturewhere experimentally the onset of a spin-gap has been obeserved.[24]R.Putz,W.Hanke,A.Muramatsu,to be published.FIGURESFIG.1.Quasiparticle dispersion along three cuts through the Brillouin zone with the high-symmetry pointsΓ=(0,0),X=(π,0),M=(π,π).The FLEX result for hole-dopingδ=0.25 and temperature T=0,1t is compared with QMC data.FIG.2.Spectral-weight function A(k,ω)for excitation energies near the chemical potential and k-vectors along high-symmetry lines in the BZ.FIG.3.Quasiparticle dispersion along high-symmetry lines in the BZ.Hartree-Fock(HF)re-sults(dashed line)and FLEX calculations which include only the charge scattering channel(dotted line)and all symmetrically chosen particle-hole and particle-particle channels(full line)are com-pared to ARPES experiments(open circles:Ref.[2],full circles:Ref.[3]).FIG.4.Energy-difference E(X)−µand energy of magnetic excitationsωspin close to the AF-point as a function of doping.。
The electronic properties of graphene
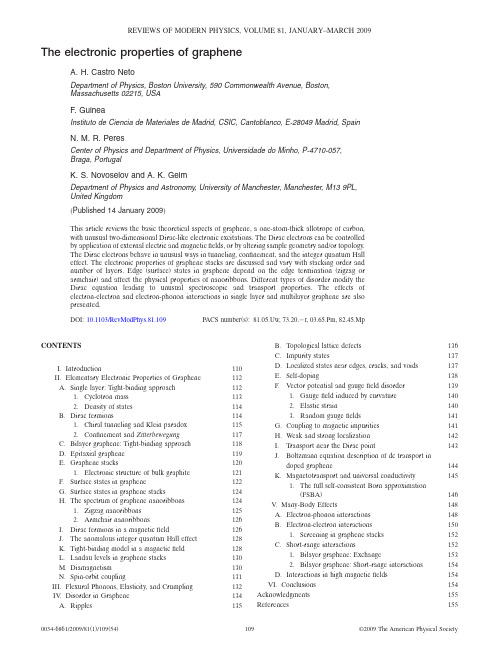
155
109
©2009 The American Physical Society
110
Edited by Foxit Reader Castro Neto et al.: The elecCtroonpicyprriogphertt(ieCs )ofbgyrapFhoenxeit Software Company,2005-2008
Graphene was eventually spotted due to the subtle op-
tical effect it creates on top of a chosen SiO2 substrate ͑Novoselov et al., 2004͒ that allows its observation with
For Evaluation Only.
efit of hindsight, no experimental tools existed to search
for one-atom-thick flakes among the pencil debris cov-
ering macroscopic areas ͑Geim and MacDonald, 2007͒.
REVIEWS OF MODERN PHYSICS, VOLUME 81, JANUARY–MARCH 2009
The electronic properties of graphene
A. H. Castro Neto Department of Physics, Boston University, 590 Commonwealth Avenue, Boston, Massachusetts 02215, USA
d带中心与配位理论ppt课件

台阶图
Fig2. Standard free energy diagram for the OER at zero potential (U=0)
Man I C, Su H Y, Calle‐Vallejo F, et al. Universality in oxygen evolution electrocatalysis on oxide surfaces[J]. ChemCatChem, 2011, 3(7): 1159-1165.
Calle-Vallejo F, Loffreda D, Koper M T M, et al. Introducing structural sensitivity into adsorption–energy scaling relations by means of coordination numbers[J]. Nature chemistry, 2015, 7(5): 403-410.
Байду номын сангаас Thank You
吉布斯自由能变
Man I C, Su H Y, Calle‐Vallejo F, et al. Universality in oxygen evolution electrocatalysis on oxide surfaces[J]. ChemCatChem, 2011, 3(7): 1159-1165.
Calle‐Vallejo F, Martínez J I, García‐Lastra J M, et al. Fast prediction of adsorption properties for platinum nanocatalysts with generalized coordination numbers[J]. Angewandte Chemie International Edition, 2014, 53(32): 8316-8319.
CdZnTe Background Measurement at Balloon Altitudes
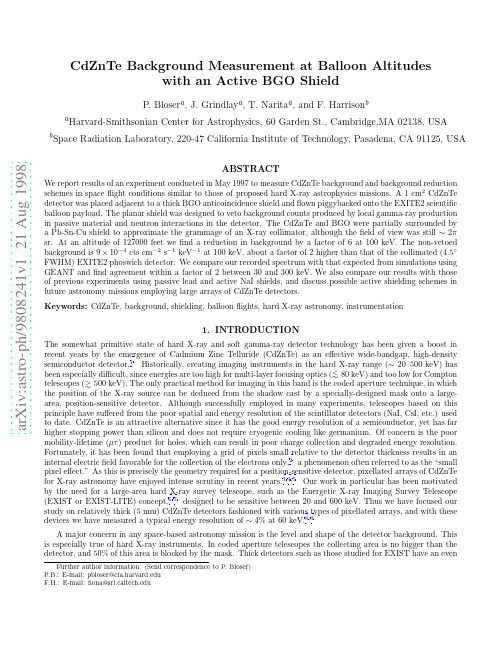
a rXiv:as tr o-ph/988241v121Aug1998CdZnTe Background Measurement at Balloon Altitudes with an Active BGO Shield P.Bloser a ,J.Grindlay a ,T.Narita a ,and F.Harrison b a Harvard-Smithsonian Center for Astrophysics,60Garden St.,Cambridge,MA 02138,USA b Space Radiation Laboratory,220-47California Institute of Technology,Pasadena,CA 91125,USA ABSTRACT We report results of an experiment conducted in May 1997to measure CdZnTe background and background reduction schemes in space flight conditions similar to those of proposed hard X-ray astrophysics missions.A 1cm 2CdZnTe detector was placed adjacent to a thick BGO anticoincidence shield and flown piggybacked onto the EXITE2scientific balloon payload.The planar shield was designed to veto background counts produced by local gamma-ray production in passive material and neutron interactions in the detector.The CdZnTe and BGO were partially surrounded by a Pb-Sn-Cu shield to approximate the grammage of an X-ray collimator,although the field of view was still ∼2πsr.At an altitude of 127000feet we find a reduction in background by a factor of 6at 100keV.The non-vetoed background is 9×10−4cts cm −2s −1keV −1at 100keV,about a factor of 2higher than that of the collimated (4.5◦FWHM)EXITE2phoswich detector.We compare our recorded spectrum with that expected from simulations using GEANT and find agreement within a factor of 2between 30and 300keV.We also compare our results with those of previous experiments using passive lead and active NaI shields,and discuss possible active shielding schemes in future astronomy missions employing large arrays of CdZnTe detectors.Keywords:CdZnTe,background,shielding,balloon flights,hard X-ray astronomy,instrumentation 1.INTRODUCTION The somewhat primitive state of hard X-ray and soft gamma-ray detector technology has been given a boost in recent years by the emergence of Cadmium Zinc Telluride (CdZnTe)as an effective wide-bandgap,high-density semiconductor detector.1Historically,creating imaging instruments in the hard X-ray range (∼20–500keV)has been especially difficult,since energies are too high for multi-layer focusing optics (<∼80keV)and too low for Compton telescopes (>∼500keV).The only practical method for imaging in this band is the coded aperture technique,in which the position of the X-ray source can be deduced from the shadow cast by a specially-designed mask onto a large-area,position-sensitive detector.Although successfully employed in many experiments,telescopes based on this principle have suffered from the poor spatial and energy resolution of the scintillator detectors (NaI,CsI,etc.)used to date.CdZnTe is an attractive alternative since it has the good energy resolution of a semiconductor,yet has farhigher stopping power than silicon and does not require cryogenic cooling like germanium.Of concern is the poor mobility-lifetime (µτ)product for holes,which can result in poor charge collection and degraded energy resolution.Fortunately,it has been found that employing a grid of pixels small relative to the detector thickness results in an internal electric field favorable for the collection of the electrons only,2a phenomenon often referred to as the “small pixel effect.”As this is precisely the geometry required for a position-sensitive detector,pixellated arrays of CdZnTe for X-ray astronomy have enjoyed intense scrutiny in recent years.3,4,5Our work in particular has been motivated by the need for a large-area hard X-ray survey telescope,such as the Energetic X-ray Imaging Survey Telescope (EXIST or EXIST-LITE)concept,6,7designed to be sensitive between 20and 600keV.Thus we have focused our study on relatively thick (5mm)CdZnTe detectors fashioned with various types of pixellated arrays,and with these devices we have measured a typical energy resolution of ∼4%at 60keV.8,9A major concern in any space-based astronomy mission is the level and shape of the detector background.This is especially true of hard X-ray instruments.In coded aperture telescopes the collecting area is no bigger than the detector,and 50%of this area is blocked by the mask.Thick detectors such as those studied for EXIST have an evenPMT, HV, Electronics BGO CdZnTePb-Sn-Cu ShieldPb-Sn ShieldAl Pressure VesselPlugFigure 1.Schematic of the CfA-Caltech CdZnTe/BGO detector.The CdZnTe is 1cm 2by 2mm thick,while the BGO is 8.2cm diameter ×6.5cm thick.The detector is shielded with Pb-Sn-Cu on the front to approximate the grammage of a passive collimator.more unfavorable ratio of detector volume to collecting area.These considerations are combined with the fact that astrophysical sources are quite weak above ∼20keV,and that the detector is placed in the high-radiation environment of the upper atmosphere or low Earth orbit.The result is that nearly all hard X-ray astronomy observations are completely background dominated,and keeping the background to a minimum is essential for obtaining high signal-to-noise.The physical processes that produce the primary background components in an instrument are highly dependent on the specific materials used in the detector and the surrounding structures.Typically the background in balloon payloads is due to the combination of diffuse cosmic gamma-rays with gamma-ray photons and energetic particles resulting from cosmic ray interactions in the atmosphere and in the payload itself.Designing effective shielding techniques is thus dependent on a thorough understanding of the interaction processes that dominate when the detector and shields are bombarded with gamma-ray photons,electrons,neutrons,and protons.Much work has been done to measure and characterize the background components in heavily-used detector materials such as NaI and CsI scintillators 10,11,12and germanium semiconductors.13A similar effort is required for CdZnTe if its potential as a detector material for astronomy is to be realized.Two measurements of CdZnTe background at balloon altitudes are described in the literature,one each by groups at Goddard Space Flight Center (GSFC)14and Caltech,15though others exist.16These experiments,described in more detail in Sect.2,employed simple passive and active shielding schemes and represent the first attempts to measure and model the basic physical processes involved.In this work we describe the first experiment designed to measure the in-flight background in a CdZnTe detector in a configuration approximating a real hard X-ray telescope.2.EXPERIMENTAL CONFIGURATIONAs a collaboration between the Harvard-Smithsonian Center for Astrophysics (CfA)and Caltech we have constructed,tested,and flown a single-element CdZnTe detector placed adjacent to a thick BGO anticoincidence shield as a piggyback experiment on the second Energetic X-ray Imaging Telescope Experiment (EXITE2)17balloon payload.The design of the instrument was motivated both by the results of previous CdZnTe balloon flights by GSFC 14and Caltech,15and by the requirements of an actual hard X-ray telescope.The flight of the GSFC CdZnTe experiment PoRTIA,flown in 1995as a piggyback on the GRIS payload and containing both a thin (2mm)and thick (5mm)CdZnTe detector,revealed a background far higher than that of a Ge spectrometer flown alongside.14This background was markedly reduced,however,when the CdZnTe detector was surrounded by NaI active shielding.Since a real astronomical instrument cannot completely surround the detector with NaI,the current experiment places the CdZnTe detector directly in front of a thick BGO crystal.The Caltech CdZnTe detector,flown on the GRIP-2payload,was passively shielded only and showed a high background which has been successfully modeled as gamma-rays produced in the passive material by cosmic ray interactions.15Proposed hard X-ray telescopes using CdZnTe,such as EXIST,6usually require some passive material near the detector in the form of a collimator;thus,such material was included in the instrument (although for the balloon-borne EXIST-LITE concept 7an active collimator may achieve lower background).Figure1shows a schematic of the CdZnTe/BGO detector,as assembled by Caltech.The detector is housed inan aluminum pressure vessel with an entrance window20mil thick.The pressure vessel wasfilled with nitrogen at ∼1atmosphere pressure.The CdZnTe detector was supplied by eV Products and is a planar,single-element device 10mm×10mm×2mm.It is centered about4mm in front of the BGO,which was supplied by JPL and is8.2cm in diameter×6.5cm thick.The BGO thus subtends∼90%of the2πsteradians behind the CdZnTe.The CdZnTe is shielded in the front by1.8mm of Pb+0.85mm of Sn+1.2mm of Cu to approximate the grammage of a graded X-ray collimator.A hole was left in the shield to allow calibration X-ray sources to shine in;this hole was covered by a Pb-Sn-Cu plug attached to the outside of the Al entrance window duringflight.The CdZnTe and BGO are shielded on the sides by1.6mm of Pb+0.9mm of Sn.Within the pressure vessel,behind the BGO,are the readout PMT,high voltage supply biasing the CdZnTe at300V,and an eV Products550preamp.The detector is connected to an electronics box designed and built at CfA containing shaping amps(shaping time∼3.3µs)for the CdZnTe and BGO signals,discriminators,and12-bit ADCs.This box also provided an interface to the mainflight computer,which read the CdZnTe/BGO data into the EXITE2payload’s data stream.The CdZnTe/BGO detector was mounted duringflight on the side of the EXITE2gondola∼1.5m above the primary phoswich detector with the thin Al window pointed straight up.The CdZnTe/BGO detector was extensively tested and calibrated in the lab at CfA prior toflight using241Amand133Ba radioactive sources.We determined the energy range to be∼15–500keV with an energy resolution (FWHM)of6.2keV at60keV(10.3%).These values were quite stable over periods of time longer than a normal balloonflight(>∼24hours).The threshold of the BGO shield discriminator was calibrated and set at∼30keV with an uncertainty of20%.The discriminator is not sharp,but rolls offover∼10keV.3.FLIGHT RESULTSThe EXITE2balloon payload,carrying the CdZnTe/BGO detector,was launched from Ft.Sumner,NM at UT 16:15,May7,1997.Higher than expected winds forced theflight to be terminated after14hours atfloat altitudes. The altitude varied between113000and127000feet during the night,corresponding to overlying atmospheric levels between∼6g cm−2and∼3.5g cm−2.Theflight computer system behaved erratically early in theflight,but performed quite well during the second half,and∼6000seconds of good CdZnTe data were collected at the highest altitudes with an average atmospheric grammage of3.55g cm−2,as well as∼4100seconds at5.1g cm−2and∼7300 seconds at6g cm−2.After theflight the CdZnTe detector was re-tested in the lab,and the energy calibration was found to be the same as before launch.Thus we believe the detector performance was quite stable during theflight.Figure2shows the EXITE2CdZnTe spectrum recorded from6006seconds of data at an average grammage of3.55 g cm−2,corresponding to an average altitude of127423feet.Both the total events and the“good,”or non-vetoed, events are plotted.The good event rate at100keV is∼9×10−4cts cm−2s−1keV−1,roughly midway between the totally actively-shielded GSFC(PoRTIA)spectrum14and the totally passively-shielded Caltech(GRIP-2)spectrum15 (see Fig.6).The uncertainty in this background level due to data telemetry throughput uncertainties is less than 5%.The good event rate is a factor of6lower than the total event rate at100keV,showing that the planar BGO shield placed behind the CdZnTe is an effective means of reducing background.The spectra recorded at5.1g cm−2 and6g cm−2are essentially identical to the spectrum in Fig.2at3.55g cm−2,ruling out significant effects due to atmospheric depth or time-dependent activation.The EXITE2CdZnTeflight spectrum will be compared in detail to the PoRTIA and GRIP-2spectra in Sect.5.4.BACKGROUND SIMULATIONSWe have simulated the background recorded by the EXITE2CdZnTe/BGO detector at3.55g cm−2using the CERN Program Library simulation package GEANT.We were able to re-create the geometry of the detector quite accurately,using cylinders,tubes,and boxes of the appropriate materials.(Figure1,in fact,was produced by GEANT.)Simulations were run recording the energy deposited in both the CdZnTe and the BGO so that both the total and non-vetoed spectra could be reproduced.In this section we detail our simulations and present results. 4.1.Simulating CdZnTe ResponseIn order to simulate accurately the spectrum recorded in the detector,we had to take into account the response of CdZnTe,specifically charge trapping.Charge is lost from an event when the charge carriers fall into deep traps within the crystal,resulting in low energy tails on recorded spectral lines.The effects of charge trapping on the measured100Energy (keV)0.00010.00100.0100C o u n t s c m -2 s -1 k e V -1All CountsNon-Rejected CountsMean Altitude = 127423. FeetMean Grammage = 3.55 g cm -2Figure 2.Spectrum recorded by the EXITE2CdZnTe/BGO detector during flight at an average atmospheric overburden of 3.55g cm −2.Both total events and non-vetoed events are shown.The non-vetoed event rate is 9×10−4cts cm −2s −1keV −1at 100keV,which is a factor of ∼6below the total rate.pulse height are described by the Hecht equation,18which relates the amount of charge collected at the electrodes of a semiconductor device to the depth at which the charge carriers are created,the electric field in the detector,and the mobility-lifetime products for the holes (µh τh )and electrons (µe τe ).Thus we record all the positions at which energy is deposited in the CdZnTe detector during an event,scale each energy according to the Hecht relation,and add up the scaled energies to give the total effective recorded energy for that event.The resulting spectra are then convolved with the detector energy resolution,taken to be a Gaussian with a width of the form FWHM =6.2(E/60)1/2keV.The electron mobility and lifetime in CdZnTe have been measured quite accurately in the recent literature,19and we adopt a typical value of µe τe =5×10−3cm 2V −1.The hole parameters are much harder to determine since the holes are so easily trapped.Until recently,values in the literature for µh τh were roughly a factor of 10less than those for µe τe .20Using µh τh =5×10−4cm 2V −1,however,produced simulated spectra that did not show the amount of low energy tailing seen in measured spectra.We determined the best value of µh τh to use by comparing the photopeak efficiencies of the simulated spectra with those of the measured spectra.We fit the X-ray line spectra with a combination of a Gaussian plus an exponential low energy tail,and defined the photopeak efficiency as the ratio of the number of counts under the Gaussian to the total number of counts.8,9Using this method we find reasonable agreement for µh τh =3×10−5cm 2V −1.We show typical examples in Fig.3,comparing recorded 241Am and 57Co spectra to the simulations.For the 60keV line from 241Am we find photopeak efficiencies of 76%for both the recorded spectrum and the simulated spectrum,while for the 122keV line from 57Co we find recorded and simulated efficiencies of 29%and 26%,respectively.We note that such low photopeak efficiencies are the result of this detector being a single-element device;a pixellated detector operating in the “small pixel”regime (a 5mm thick detector with 1.5mm pixels)gives an efficiency at 60keV of ∼90%,as we have reported elsewhere.8,9Such a low value of µh τh has been found elsewhere when comparing simulated charge transport to measurements of CdZnTe.214.2.Background ComponentsIn this section we describe the various components to the CdZnTe/BGO instrument background that we simulate in our GEANT code.Background is produced by cosmic ray interactions in the atmosphere and payload material,generating both gamma-rays and energetic particles.4.2.1.Shield Leakage from Atmospheric and Cosmic Diffuse Gamma-RaysInstruments at balloon altitudes are irradiated by gamma-rays from the diffuse cosmic X-ray and gamma-ray back-grounds and from the atmosphere.The atmospheric gamma-rays are produced mainly by bremsstrahlung from100200300400500600700Channel 0100200300400500600C o u n t s A 020406080KeV 050010001500200025003000C o u n t s B050010001500Channel 050100150200250C o u n t s C 050100150200KeV 02004006008001000C o u n t s D Figure parison of recorded and simulated CdZnTe spectra.A)Recorded 60keV spectrum from 241Am.B)Simulated 241Am spectrum.C)Recorded 122keV and 136keV 57Co spectrum.D)Simulated 57Co spectrum.Here µe τe =5×10−3cm 2V −1and µh τh =3×10−5cm 2V −1.electrons which are in turn the result of cosmic ray interactions (the decay of charged mesons or the decay of neu-tral pions followed by pair production).These gamma-rays can penetrate shielding and interact in the detector or surrounding material by photoelectric absorption,Compton scattering,and pair production.All of these processes can generate background counts in the detector,and this we refer to as “shield leakage.”The total cosmic and atmospheric gamma-ray flux at balloon altitudes has been measured by many experiments and summarized in a paper by Gehrels.13We adopt the Gehrels parameterization at 3.5g cm −2as the input spectrum to our GEANT simulations.This spectrum depends on zenith angle,slightly increasing in flux for upward-going gamma-rays since much of the production occurs in the air below the payload.The parameterization is uncertain by a factor of 1.4-2,especially above a few 100keV.15A parameterization is also given for a depth of 5g cm −2,but it differs from the 3.5g cm −2spectrum by only ∼20%,and then only at 10MeV,where the uncertainties approach a factor of 2.We therefore do not expect the recorded spectrum at 5g cm −2to differ significantly from the spectrum at 3.5g cm −2,and this agrees with our observations (see Sect.3).We restrict our modeling to the 3.5g cm −2case for simplicity,however.The gamma-ray photons are introduced on the outside of the aluminum pressure vessel and allowed to interact via the processes listed above in all the materials in the detector and surrounding structures.The total energy deposited in both the CdZnTe and the BGO for each event are finally recorded.4.2.2.Locally Produced Gamma-RaysThe same processes that produce gamma-rays in the atmosphere also generate photons in the payload materials,namely bremsstrahlung of electrons from the atmosphere as well as secondary electrons produced in the payload mass by cosmic rays.(Electrons generated in the payload by external gamma-rays are included in the shield leakage component,described above.)Interactions in active shielding materials will generally be self-vetoed,but gamma-ray production in passive materials will contribute to the background as long as the path of the primary electron through the passive material does not also take it through an active shield.Since the electron fluxes at balloon altitudes are poorly-determined and it is difficult to calculate their production by cosmic ray interactions in the payload,we adopt the common practice of assuming that the gamma-ray production by electrons in different materials is thesame as that at a given depth in the atmosphere,with a normalization depending on the material’s atomic number.We use the gamma-ray production per unit mass of air given by Dean22and scale it according to the material’s radiation length in g cm−2.15The photons are then produced throughout the passive materials in the detector andpropagated using GEANT as before.In order to reproduce both the total and the non-vetoed spectra,a geometriccorrection is applied to reduce the non-vetoed counts by the fraction of solid angle at the passive material that is subtended by the BGO.The main EXITE2phoswich detector was approximated as an85kg block of aluminum1.5m below the pressure vessel to see whether local production in the gondola structures contributed to the background.4.2.3.Neutron-Induced BackgroundAnother product of cosmic ray interactions in the atmosphere and in the payload is aflux of neutrons that may interact in the detector due to inelastic scattering or nuclear reactions.The background due to elastic scattering hasbeen found to be negligible in CdZnTe due to the high atomic weight of Cd and Te.15Neutron effects become moreimportant in experiments using thick active shielding,since the gamma-ray contributions are effectively reduced. Since they are neutral particles,neutrons may penetrate such shielding without generating a veto.The nuclear(n,γ)reaction has a high cross section in Cd for thermal neutrons below0.4eV:the nucleus absorbs a neutron and is excitedto∼8MeV(the neutron binding energy)above the ground state.The nucleus de-excites immediately,emitting ∼4gamma-rays which may then Compton scatter in the CdZnTe.The thermal neutronflux from the atmosphere is small(roughly10−3neutrons cm−2s−1at0.5eV,23which,if each produces4gamma-rays at2000keV that Compton scatter into aflat continuum below this energy,will generate only∼2×10−7cts cm−2s−1keV−1in thedetector),and so we do not expect the(n,γ)reaction to contribute much to the detector background.If,however,there were a local source of thermal neutrons,this reaction might become important.We discuss this,as well as a crude measurement we made on thisflight of atmospheric neutronfluxes,in a forthcoming paper.Inelastic scattering excites the nucleus of the target atom through the(n,n′,γ)reaction,and∼3de-excitation photons are emitted with mean energies lower than those generated in the(n,γ)reaction.Both of these reactions emit“prompt”gamma-rays which may be vetoed by an active shield.Radioactive isotopes may be produced by nuclear reactions(“activation”) which result in delayed gamma-rays that cannot be vetoed by active shielding;although this component has been studied previously,15it is complicated and not a major factor below several hundred keV,so we neglect it here.The neutron contribution to the background is difficult to estimate accurately,and since previous attempts to model CdZnTeflight data with active shielding using neutron calculations have proved difficult,15we do not attempt to calculate this component in detail here.Rather,we compare our gamma-ray calculations to the observed spectrum to see whether neutron effects can reasonably be responsible for the difference.4.3.Simulation ResultsThe results of our GEANT simulations of the gamma-ray components of the CdZnTe/BGO detector background are shown in Fig.4,compared to the actual measurements,between20and300keV.Fig.4a shows the“good,”or non-vetoed spectra,and Fig.4b shows the total spectra.A CdZnTe event was considered to be vetoed if more than 30keV was deposited anywhere in the BGO.The contributions from shield leakage and local gamma-ray production are shown separately.The non-vetoed background(Fig.4a)is dominated by shield leakage,and the predicted level agrees well(within a factor of2)with the data between30and300keV.There is clearly an excess in the data below30keV compared to the simulation.Above∼100keV the simulated spectrum seems to fall offmore rapidly than the measured spectrum,but at300keV they are still within a factor of2of each other.There is a small peak in the predicted shield leakage spectrum near70keV,presumably due to Pbfluorescence.Though not discernible in the data,this indicates that Compton and photoelectric interactions of the primary gamma-rays in the passive material are contributing to the background.The total background predicted(Fig.4b)is well within a factor of2of that measured between30and100keV,and then it too falls offmore rapidly than the recorded spectrum.Shield leakage and local gamma-ray production have roughly equal magnitudes throughout the energy range shown,with local production starting to dominate at low energies.There is also evidence of a low energy excess in the data compared to the simulation.It appears that the total background has been underestimated by gamma-ray effects alone,especially above100keV.The simulated total spectrum is only a factor of∼2greater than the simulated good spectrum at100keV,as opposed to a factor of∼6difference in the data.Wefind that local production in the main EXITE2phoswich detector does not produce a significant number of counts in the CdZnTe,since it subtends a small solid angle and is below the BGO.100Energy (keV)0.00010.0010C o u n t s c m -2 s -1 k e V -1Total Simulated "Good" Background Shield Leakage Local Gamma-RaysMeasured "Good" Background A100Energy (keV)0.00010.00100.0100C o u n t s c m -2 s -1 k e V -1Total Simulated Background Shield Leakage Local Gamma-Rays Measured Total BackgroundBFigure parison of the simulated spectra to the measured flight spectra for the EXITE2CdZnTe/BGO detector.A)“Good”events (not vetoed by the BGO).B)All events.The contributions from shield leakage and local gamma-rays are indicated by the dotted and dashed lines,respectively.5.DISCUSSION AND CONCLUSIONSThe rough agreement between the simulations and the flight CdZnTe/BGO data is encouraging:the measured and simulated good spectra lie within a factor of 2of each other below 300keV.Considering that the input atmospheric spectra are uncertain by factors approaching 2,especially at higher energies,this agreement is satisfactory.The results indicate that local gamma-ray production in the passive material has been effectively vetoed even by such a simple active shielding configuration.With more care,this component should be rendered completely negligible in future instruments.An active collimator,as suggested for the EXIST-LITE concept,7should further reduce this source of background,as well as eliminate the contributions from fluorescence and Compton scattering.The factor of 6reduction in background achieved by BGO tagging is not reproduced by the simulations.This,together with the excesses at high and low energies seen in the data,and the fact that the total background is under-estimated,indicates that additional components are present in the CdZnTe background that are not accounted for by gamma-ray interactions alone.These components are effectively rejected by vetoes from the BGO shield,although there is a low-energy excess seen in the good events spectrum as well.It is difficult to attribute unambiguously this good-event low-energy excess to additional background components,however,because the excess occurs near the ∼10keV-wide threshold of the BGO shield:low energy events that deposit little energy in the shield are less likely to be vetoed,resulting in an artificial excess in the “good”event spectrum.No such effects enter into the total spectrum,however.In Fig.5we show the difference between the total recorded and simulated spectra from Fig.4b.Although this difference is highly uncertain,there is evidence of an excess below 40keV,as well as between ∼100and 200keV.The most obvious explanation for the difference between the simulated gamma-induced spectrum and the data is provided by neutron interactions.Detailed calculations of the neutron-induced background in CdZnTe detectors in various configurations do indeed indicate that prompt neutron interactions ((n,γ),(n,n ′,γ))contribute to the background below about 50keV 15;however,this background is always at a level far lower than that indicated by Fig.5(∼10−4cts cm −2s −1keV −1at 20keV,as opposed to ∼10−2cts cm −2s −1keV −1in the figure).As shown in Sect.4.2.3,the thermal component is expected to be completely negligible unless a bright source of thermal neutrons other than the atmosphere is present.Radioactive decay of the products of neutron activation can contribute to the background up to many hundreds of keV,but again at a level far below what is seen here.It is possible,however,that radioactive decay of activation products in the BGO shield itself is producing a large high energy background in the CdZnTe.This background would certainly be efficiently vetoed.It remains to be seen whether more detailed estimates of the neutron contribution to the CdZnTe/BGO detector background can provide an explanation for the large measured background and its marked reduction with the BGO shield.。
AMPS-1D manual
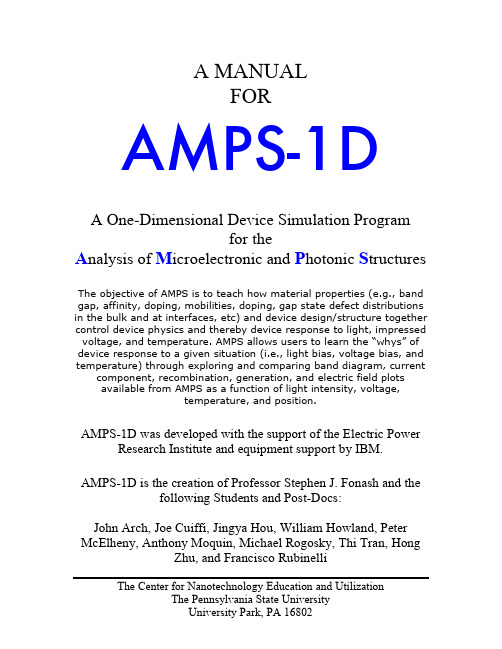
The Center for Nanotechnology Education and UtilizationThe Pennsylvania State UniversityA MANUALFORAMPS-1DA One-Dimensional Device Simulation Programfor theA nalysis of M icroelectronic and P hotonic S tructuresThe objective of AMPS is to teach how material properties (e.g., band gap, affinity, doping, mobilities, doping, gap state defect distributions in the bulk and at interfaces, etc) and device design/structure together control device physics and thereby device response to light, impressed voltage, and temperature. AMPS allows users to learn the “whys” of device response to a given situation (i.e., light bias, voltage bias, and temperature) through exploring and comparing band diagram, current component, recombination, generation, and electric field plots available from AMPS as a function of light intensity, voltage,temperature, and position.AMPS-1D was developed with the support of the Electric Power Research Institute and equipment support by IBM.AMPS-1D is the creation of Professor Stephen J. Fonash and thefollowing Students and Post-Docs:John Arch, Joe Cuiffi, Jingya Hou, William Howland, Peter McElheny, Anthony Moquin, Michael Rogosky, Thi Tran, HongZhu, and Francisco RubinelliCONTENTSCONTENTS ....................................................................................................................i PREFACE.....................................................................................................................iii FIGURES......................................................................................................................iv CHAPTER 1 INTRODUCTION. (1)1.1 AMPS and Its Features (1)1.2 About This Manual (2)1.3 An Overview of How AMPS Works (3)1.4 Examples of AMPS Output (3)1.4.1 An example — a Al 0.3Ga 0.7As/GaAs Heterojunction Diode (3)1.4.2 An Example — a Triple Junction Solar Cell..............................................................................6CHAPTER 2 MATHEMATICAL MODELING & SOLUTION TECHNIQUES (10)2.0 Introduction (10)2.1 Poisson’s Equation (10)2.1.1 The Delocalized (Band) State Populations n and p (11)2.1.2 Localized (Gap) State Populations N D +, N A -, n t , and p t (13)2.1.2.1 Doping Levels (N D + and N A -) (13)2.1.2.1a Discrete Dopant Levels (N d D,i and N d A,j ) (14)2.1.2.1b Banded Dopant Levels (N b D,i and N b A,j) (16)2.1.2.1c Generalized Dopant Level Distributions (18)2.1.2.2 Defect (Structural and Impurity) Levels (n t and p t ) (18)2.1.2.2a Discrete and Banded Defect (Structural and Impurity) Levels (19)2.1.2.2b Generalized Defect (Structural and Impurity) Level Distributions (19)2.2 The Continuity Equations (20)2.2.1 Electron and Hole Current Density (20)2.2.2 The Recombination Mechanisms (21)2.2.2.1 Direct (Band-to-band) Recombination (21)2.2.2.2 Indirect (Shockley-Read-Hall) Recombination (22)2.2.3 Optical Generation Rate (23)2.2.4 Boundary Conditions (25)2.3 Solution Techniques (27)2.3.1 Discretization of the Definition Domain (27)2.3.2 Discretization of the Differential Equations (28)2.3.3 Newton-Raphson Method (30)2.4 Constructing the Full Solution.........................................................................................31CHAPTER 3 MATERIAL PARAMETERS.. (32)3.0 Introduction (32)3.1 Parameters for Representing Semiconductor Properties (32)3.1.1 Lifetime picture (32)3.1.2 DOS picture (34)3.1.2.1 Parameters for Single Crystal Semiconductor Materials (34)3.1.2.1.1 Band State Parameters (35)3.1.2.1.2 Localized (Gap) State Parameters (36)3.1.2.1.2.1 Parameters for Doping Levels (36)3.1.1.2.1a Parameters for Discrete Dopant Levels (36)3.1.2.1.2.1b Parameters for Banded Dopant Levels (37)3.1.2.1.2.2 Parameters for Defect Levels (37)3.1.2.1.2.2a Parameters for Discrete and Banded Defect Levels (37)3.1.2.1.2.2b Parameters for the Continuous Defect Levels (38)3.1.2.1.3 Parameters for Optical Properties (40)3.1.2.2 Parameters for Amorphous Semiconductor Materials (40)3.1.2.3 Parameters for Polycrystalline Semiconductor Materials (41)3.2 Parameters for Insulator Properties (41)3.3 Parameters for Metal Properties (41)3.4 Parameters for Interface Properties (42)3.5 Parameters for Materials with Spatially Varying Properties (42)CHAPTER 4 PROCEDURE FOR RUNNING AMPS (43)4. 1 Overview (43)4. 2 How to Generate Device Characteristics (43)4. 2. 1 Dark IV Characteristics (43)4. 2. 2 Light IV Characteristics (43)4. 2. 3 Spectral Response (44)4. 3 Surface Photovoltage Response (44)4.4 Procedure for Inputting Parameters (44)4.4.1 List of Input Parameters (45)4.4.1.1 Parameters that Apply to the Entire Device (45)4.4.1.2 Parameters that Apply to a Particular Region (46)4.4.1.3 Parameters that Define the Illumination Spectrum (51)4.5 Choosing A Grid (51)APPENDIX A OPTICAL GENERATION RATE (53)APPENDIX B TRIAL FUNCTION FOR THE CURRENT DENSITY (55)APPENDIX C INSTALLATION (58)C. 1 System requirement (58)C. 2 Installation Instructions (58)C. 3 Running AMPS-1D (58)C. 4 Problems and questions (59)PREFACEAMPS is a very general program for analyzing and designing transport in microelectronic and photonic structures. It differs from other transport analysis programs such as PICES in a number of ways. Among them are its ability to handle any defect and doping energy gap and special distribution, its incorporation of S-R-H and band-to-band recombination, its incorporation of Boltzmann and Fermi-Dirac statistics, its ability to handle varying material properties, its very general treatment of contacts, and its ability to handle transport in devices under voltage bias, light bias, or both.This manual for AMPS-1D is intended for those using our window ‘95/NT version. We apologize in advance for the fact that this manual will get out of date but, as we are sure you understand, AMPS is a constantly growing, developing package. However, most of what is said here will remain valid and should be useful.AMPS would not exist without the support of the Electric Power Research Institute. In particular it would not exist without the encouragement, guidance, and questioning of Dr. Terry Peterson of EPRI and without the vision of Dr. Ed DeMeo and Dr. John Crowley.Stephen FonashElectronic Materials and Processing Research LaboratoryPenn State UniversityFIGURESFIGURE 1-4. ILLUMINATED CURRENT-VOLTAGE CHARACTERISTIC AND CELL PERFORMANCE VALUES FOR THIS TRIPLE JUNCTION SOLAR CELL.7 FIGURE 1-5. BAND DIAGRAM OF THIS TRIPLE JUNCTION IN THERMODYNAMIC EQUILIBRIUM.8 FIGURE 1-6. ELECTRON AND HOLE LIFETIME AT V OC VERSUS POSITION FOR A TRIPLE.ONLY MEANINGFUL FOR REGIONS WHERE CARRIER IS THE MINORITY CARRIER.9 FIGURE 2-1. A BAND DIAGRAM OF A SCHOTTKY BARRIER IN THERMODYNAMIC EQUILIBRIUM.11 FIGURE 2-2. DENSITY OF STATES PLOT REPRESENTING DISCRETE LOCALIZED DOPANT LEVELS. THE DONOR LEVELS ARE LOCATED POSITIVELY DOWN FROM THECONDUCTION BAND AND THE ACCEPTOR LEVELS ARE LOCATED POSITIVELY UPFROM THE VALENCE BAND.14 FIGURE 2-3. DENSITY OF STATES PLOT SHOWING A BAND OF DOPANT STATES. ENERGIES FOR DONOR SITES ARE MEASURED POSITIVELY DOWN TO E1 FROM THECONDUCTION BAND AND THOSE FOR ACCEPTOR SITES ARE MEASURED POSITIVELY UP TO E1 FROM THE VALENCE BAND.16 FIGURE 2-4. DENSITY OF STATES PLOT REPRESENTING A GENERALIZED DISTRIBUTION OF DOPANT STATES.18 FIGURE 2-5. THIS FIGURE ILLUSTRATES THE PHOTON FLUX AT SOME POINT X MOVING TO THE LEFT AND THE PHOTON FLUX AT SOME POINT X MOVING TO THE RIGHT IN THE J+1 MATERIAL REGION.23 FIGURE 2-6. THIS FIGURE ILLUSTRATES THE REFLECTION OF THE PHOTON FLUX AT THE J+1 BOUNDARY AND AT THE J BOUNDARY OF THE J+1 REGION.24 FIGURE 2-7. A GRID USED IN NUMERICAL METHODS. THERE ARE N SLABS (DASHED LINES) AND N+1 MAJOR GRID POINTS (SOLID LINES). THE EXAMPLE SHOWN HERE ISA UNIFORM GRID.27 FIGURE 3-1 A BAND DIAGRAM FOR A LAYER OF A DEVICE THAT HAS CONSTANT MATERIAL PARAMETERS.35 FIGURE 3-2. URBACH TAILS ONLY.39 FIGURE 3-3. A MORE COMPLICATED DENSITY OF STATES: URBACH TAILS AND A CONSTANT MID-GAP DISTRIBUTION (CONTRIBUTIONS FROM CONSTANTDISTRIBUTION ARE IGNORED BEYOND E LO AND E UP.39 FIGURE 4-1 SCHEMATIC BAND DIAGRAM OF A SEMICONDUCTOR DEVICE UNDER AN APPLIED VOLTAGE V APP .46 FIGURE 4-2 AN EXAMPLE OF ONE DISCRETE DONOR LEVEL AND ONE DISCRETE ACCEPTOR LEVEL.47 FIGURE 4-3 “V-SHAPED” REPRESENTATION OF DENSITY OF STATES.48 FIGURE 4-4. “U-SHAPED” REPRESENTATION OF THE DENSITY OF STATES.49 FIGURE 4-5 AN EXAMPLE OF ONE GAUSSIAN DONOR LEVEL AND ONE GAUSSIAN ACCEPTOR LEVEL.50 FIGURE A-1. REFLECTION AND TRANSMISSION WITHIN A DEVICE OF FIVE REGIONS OF DIFFERING MATERIAL PARAMETERS.53 FIGURE B-1. A GRID USED IN NUMERICAL METHODS. THERE ARE N SLABS AND N+1 MAJOR GRID POINTS (REPRESENTED BY SOLID LINES). THE DASHED LINES ARE THE POINTS WHERE THE CURRENT DENSITY TRIAL FUNCTION IS SOLVED.56CHAPTER 1INTRODUCTION1.1 AMPS and Its FeaturesThis manual is an introduction to a very general, one-dimensional computer program for simulating transport physics in solid state devices. It uses the first-principles continuity and Poisson’s equations approach to analyze the transport behavior of semiconductor electronic and opto-electronic device structures. These device structures can be composed of crystalline, polycrystalline, or amorphous materials or combinations thereof. This program, called AMPS (A nalysis of M icroelectronic and P hotonic S tructures), numerically solves the three governing semiconductor device equations (the Poisson equation and the electron and hole continuity equations) without making any a-priori assumptions about the mechanisms controlling transport in these devices. With this general and exact numerical treatment, AMPS may be used to examine a variety of device structures that include• homojunction and heterojunction p-n and p-i-n, solar cells and detectors;• homojunction and heterojunction p-n, p-i-n, n-i-n, and p-i-p microelectronic structures;• multi-junction solar cell structures;• multi-junction microelectronic structures;• compositionally-graded detector and solar cell structures;• compositionally-graded microelectronic structures;• novel device microelectronic, photovoltaic, and opto-electronic structures;• Schottky barrier devices with optional back layers.From the solution provided by an AMPS simulation, output such as current voltage characteristics in the dark and, if desired, under illumination can be obtained. These may be computed as a function of temperature. For solar cell and detector structures, collection efficiencies as a function of voltage, light bias, and temperature can also be obtained. In addition, important information such as electric field distributions, free and trapped carrier populations, recombination profiles, and individual carrier current densities as a function of position can be extracted from the AMPS program. As stated earlier, AMPS’ versatility can be used to analyze transport in a wide variety of device structures that can contain combinations of crystalline, polycrystalline, or amorphous layers. AMPS is formulated to analyze, design, and optimize structures intended for microelectronic, photovoltaic, or opto-electronic applications.A comparison of AMPS with other known programs shows that AMPS is the only computer modeling program available that incorporates all of the following physics:• a contact treatment that allows thermionic emission and recombination to take place at device contacts;• a very generalized gap state model that can fit any density of states distribution in the bulk or at an interface;• both band-to-band and Shockley-Read-Hall recombination;• a recombination model that computes Shockley-Read-Hall recombination traffic through any inputted general gap state distribution instead of the often-used singlerecombination level approach;• full Fermi-Dirac, and not just Boltzmann, statistics;• gap state populations computed with actual-temperature statistics rather than the often used T=0K approach;• a trapped charge model that accounts for charge in any inputted general gap state distribution;• a gap state model that allows capture cross-sections to vary with energy;• gap state distributions whose properties can vary with position;• carrier mobility that can vary with position;• electron and hole affinities that can vary with position;• mobility gaps that can differ from optical gaps;• the ability to calculate device characteristics as a function of temperature in both forward and reverse bias as well as with or without illumination;• the ability to analyze device structures fabricated using single crystal, polycrystalline, or amorphous materials or all three.1.2 About This ManualThis manual assumes the user has completed an introductory course in semiconductor device physics and is familiar with mathematical concepts such as Poisson’s equation and the continuity equations. A working knowledge of numerical methods is helpful, but not actually required for working with the AMPS program. This manual explains the approach used in AMPS for • modeling of hole and electron transport, including a discussion of the basic equations and solution techniques (Chapter 2);• parameterizing material properties (Chapter 3)• semiconductor materials• insulators• metals• interfaces• materials with position dependent properties;• running programs to obtain band diagrams in thermodynamic equilibrium and for running programs for devices under voltage, light bias, or both (non-thermodynamicequilibrium) (Chapter 5).The manual begins by using the introductory chapter to offer a brief overview of AMPS, and to present some examples of its capabilities. Chapter 2 can be skipped but it has been included in this manual for those who are interested it discusses the physical and mathematical bases of the simulation programs. Chapter 3 discusses parameterizing material properties and it shows that close attention must be given to the particular types of materials the user intends to “build” his or her structure. The AMPS programs will ask the user to input these specific parameters. Chapter 4 describes the heart of AMPS: the procedures for obtaining the detailed physics and terminal characteristics of devices under voltage bias, light bias, or both.1.3 An Overview of How AMPS WorksIn briefly overviewing our methods of modeling microelectronic and opto-electronic devices, we first note that the physics of device transport can be captured in three governing equations: Poisson’s equation, the continuity equation for free holes, and the continuity equation for free electrons. Determining transport characteristics then becomes a task of solving these three coupled non-linear differential equations, each of which has two associated boundary conditions. In AMPS, these three coupled equations, along with the appropriate boundary conditions, are solved simultaneously to obtain a set of three unknown state variables at each point in the device: the electrostatic potential, the hole quasi-Fermi level, and the electron quasi-Fermi level. From these three state variables, the carrier concentrations, fields, currents, etc. can then be computed. To determine these state variables, the method of finite differences and the Newton-Raphson technique are incorporated by the computer. The Newton-Raphson Method iteratively finds the root of a function or roots of a set of functions if given an adequate initial guess for these roots. In AMPS, the one-dimensional device being analyzed is divided into segments by a mesh of grid points, the number of which the user decides. The three sets of unknowns are then solved for each particular grid point. We note that AMPS allows the mesh to have variable grid spacing at the discretion of the user. As noted, once these three state variables are obtained as a function of x, the band edges, electric field, trapped charge, carrier populations, current densities, recombination profiles, and any other transport information may be obtained.1.4 Examples of AMPS OutputThe following examples illustrate the different types of semiconductor structures that AMPS can simulate and also give a sampling of the output information AMPS can generate. These are just two straight-forward examples intended to give the reader some indication of the power and versatility of AMPS.1.4.1 An example — a Al0.3Ga0.7As/GaAs Heterojunction DiodeFigures 1-1. and 1-2. give the room-temperature current-voltage characteristic in forward and reverse bias and the band structure in thermodynamic equilibrium for an Al0.3Ga0.7As/GaAs p-n heterojunction diode. The doping happens to have been taken to be 1016 cm-3 in both layers.Figure1-1 Current-Voltage characteristic in forward and reverse bias.Figure1-2 Band structure in thermodynamic equilibrium.Figure 1-3. shows the space charge at -1, 0, and +1 volts (i.e., forward, zero, and reverse biases, respectively). This example demonstrates how AMPS can be used to determine the amount of charge transfer in the space charge regions of heterojunction structures and the widths of these space charge layers as a function of bias. The current-voltage characteristic, along with other output from AMPS, can be used to determine how different transport mechanisms become important at different magnitudes of forward and reverse bias.Figure 1-3 Spatial dependence of the electric field at three different bias voltages.-1V, 0V, 1V.1.4.2 An Example — a Triple Junction Solar CellFigure 1-4. gives the illuminated current-voltage characteristic and the cell performance values obtained from AMPS simulation of an a triple p-i-n solar cell. The density of states used to model the a-Si:H materials consists of exponential tail states and midgap states. Fig 1-5. shows the band diagram of this complicated cell in thermodynamic equilibrium. Figure 1-6 shows the electron and hole lifetime at open circuit voltage. This example illustrates AMPS usefulness in determining the transport mechanisms controlling cell performance and in optimizing cell design. In addition, this final example also highlights the versatility of AMPS by demonstrating its ability to modelcomplicated structures with many layers of different materials.Figure 1-4. Illuminated current-voltage characteristic and cell performance values for this triple junction solar cell.Figure 1-5. Band diagram of this triple junction in thermodynamic equilibrium.Figure 1-6. Electron and hole lifetime at V OC versus position for a triple. Only meaningful for regions where carrier is the minority carrier.CHAPTER 2MA THEMA TICAL MODELING & SOLUTION TECHNIQUES 2.0 IntroductionAs noted in Chapter 1, this chapter may be skipped. It is intended for those who want to “open AMPS up and get an idea how it ticks.”Understanding of how AMPS “ticks” begins by noting that with the continuum approach used in AMPS, the physics of device transport can be captured in three governing equations: Poisson’s equation, the continuity equation for free holes, and the continuity equation for free electrons.Determining transport characteristics then becomes a task of solving these three coupled non-linear differential equations subject to appropriate boundary conditions. These three equations and the corresponding boundary conditions, along with the numerical solution technique used to solve them, will then be the subject of this chapter.We assume in AMPS that the material system under examination is in steady state. That is, it is assumed that there is no time dependence. It follows that the terminal characteristics generated by AMPS are the quasi-static characteristics.2.1 Poisson’s EquationPoisson’s equation links free carrier populations, trapped charge populations, and ionized dopant populations to the electrostatic field present in a material system. In one-dimensional space,Poisson’s equation is given byd dx !"#$%&-'(x)d (’dx = q •[p(x)-n(x)+N D +(x)-N A -(x)+p t (x)-n t (x)]where the electrostatic potential (' and the free electron n, free hole p, trapped electron n t , and trapped hole p t , as well as the ionized donor-like doping N D + and ionized acceptor-like doping N A -concentrations are all functions of the position coordinate x. Here, ' is the permittivity and q is the magnitude of the charge of an electron.Since band diagrams show the energies allowed to electrons and since the electrostatic potential ('is defined for a unit positive particle, the use of (' in the above equation can be inconvenient. The local vacuum level E VL , which is the top or escape energy of the conduction band, varies only due to the presence of an electrostatic field [1]. Its derivative, therefore, is proportional to the electrostatic field ). In fact, if we remember to measure the position of the local vacuum level from a reference using the quantity ( measured in eV, then ) = d (/dx. As seen in Fig. 2-1, AMPS uses ( not (' and always chooses the reference for ( to be the position of the local vacuum level in the contact at the right hand side of any general device structure. With this particular example in Fig.2-1 of a Schottky barrier (, as we have defined it, is seen to be a negative quantity in much of then + back-contact layer and a positive quantity essentially through the remainder of the ing this way of locating the local vacuum level and remembering that its spatial derivative is the electrostatic field allows us to rewrite Poisson’s equation in terms of the local vacuum level (measured in eV. This givesd dx !"#$%&'(x)d (dx = q •[p(x)-n(x)+N D +(x)-N A -(x)+p t (x)-n t (x)](2.1)Equation (2.1) is the form of Poisson’s equation that AMPS uses.Having settled on a formulation of Poisson’s equation that will be convenient, we now realize that AMPS needs expressions for the six new dependent variables n, p, n t , p t , N D +, and N A - introduced in Equation 2.1.E FE F E C E V E G *ex=Lx=0n+layer +bo +bL (E VL...Figure 2-1. A band diagram of a Schottky barrier in thermodynamic equilibrium.2.1.1 The Delocalized (Band) State Populations n and pAssuming that a parabolic relation between the density of states N(E) of the delocalized states of the bands and the energy E - measured positively moving away from either band edge - exists such that N(E) , E 1/2, the free carrier concentrations in thermodynamic equilibrium or under voltage bias, light bias, or both are computed in AMPS using the general expressions [2]n = N c F 1/2 exp !#$&E F -E c kT (2.1.1a)p = N v F 1/2 exp !#$&E V -E F kT (2.1.1b)These general expressions allow for the possibility of degeneracy; i.e. AMPS includes both Fermi-Dirac and Boltzmann statistics. In these expressions N c and N v are the band effective densities of states for the conduction and valence bands, respectively. In AMPS these are user chosen material parameters. For crystalline materials they are given by [2]N c = 2!"#$%&2-m n *kT h 2 3/2 (2.1.1c)N v = 2!"#$%&2-m p *kT h 2 3/2 (2.1.1d)where m n * is the electron effective mass, m p * is the hole effective mass, k is the Boltzmann constant, and h is Planck’s constant.The Fermi integral of order one-half appearing in Equation 2.1.1a and b is defined as [6]F 1/2(.)= 2-E E 1+ exp(E -)1/2d .0/0 (2.1.1e)E 1/2 where . - the Fermi integral argument - is expressed as.n = !"#$%&E F -E c kT (2.1.1f)for free electrons and .p = !"#$%&E v -E F kT (2.1.1g)for free holes. We note that for .n > 3 or .p > 3, the function F 1/2 reduces to the corresponding Boltzmann factorsexp !#$&E F -E c kT (2.1.1h)or exp !#$&E v -E F kT (2.1.1i)In our formulation of AMPS we have chosen to write n and p in terms of Boltzmann factors yet to allow the possibility of degeneracy and the need for Fermi-Dirac statistics. To do this we define the Fermi-Dirac degeneracy factor 1 as1n = F 1/2(.n )exp(.n ) (2.1.1j)for free electrons and as1p = F 1/2(.p )exp(.p ) (2.1.1k)for free holes. With these definitions Equations 2.1.1a and 2.1.1b becomen = N c 1n exp(.n ) (2.1.1l)p = N v 1n exp(.p )(2.1.1m)which are valid for degenerate as well as non-degenerate situations.When a device is driven out of thermodynamic equilibrium by a voltage bias, a light bias, or both the quantities n and p can still be computed using Equations 2.1.1a - 2.1.1e. It is only necessary to replace the equilibrium Fermi-level E F with the quasi-Fermi level E F n in Equation 2.1.1a and the quasi-Fermi level E F p in Equation 2.1.1b. This is what AMPS does in going from thermodynamic equilibrium to cases with voltage bias, light bias, or both.2.1.2 Localized (Gap) State Populations N D +, N A -, n t , and p tHaving obtained expressions for the n and p terms appearing in Poisson’s equation, we must now develop expressions for the other quantities contributing to the development of charge. Since we have accounted for all the free charge, any additional charge must be in gap states.In general there may be a variety of different types of gap (i.e., localized) states existing in the energy gap of a semiconductor or insulator. AMPS breaks these into states that are inadvertently present due to defects and impurities and into states that are purposefully present due to doping.There may be donor-like and acceptor-like states among both classes. There may also be states that are continuously distributed in energy or discretely distributed in energy in both classes.AMPS allows for different distributions of these states at interfaces and at different places in the bulk material.In the case of the gap states which are not purposefully present, but are due to defects and impurities, AMPS defines n t as being the number of charged acceptor-like sites per volume (i.e.,trapped electrons) and p t as being the number of charged donor-like sites per volume (i.e., trapped holes) in this class of states. In the case of the gap states which are purposefully present due to doping AMPS defines N A - as being the number of ionized acceptor-dopant sites per volume.Correspondingly N D + is defined as being the number of ionized donor-dopant sites per volume.2.1.2.1 Doping Levels (N D + and N A -)We turn first to the charge residing in localized doping levels. The doping levels in our usage include gap states which are characterized by discrete levels and gap states that form a band with a bandwidth defined by an upper energy boundary and a lower energy boundary. This latter case of localized gap state bands can arise if heavy doping is present in a structure. It is important to note that any combination of these two unique types of states is acceptable to AMPS (see section2.1.2.1c). In any case, the total charge arising in these states can be represented byN D + = N d D + + N b D +(2.1.2.1a)for the donor-dopant levels andN A - = N d A - + N b A - (2.1.2.1b)for the acceptor-dopant levels. Here, N D + and N A - seen in Poisson’s equation (Equation 2.1), are the total charges arising from both the discrete and banded dopant energy levels. In these equationsN d D + and N d A - represent the total charge originating from discrete donor and acceptor concentrations, respectively, while N b D + and N b A - represent the total charge developed by any banded donor and acceptor levels, respectively.2.1.2.1a Discrete Dopant Levels (N d D,i and N d A,j )Discrete localized dopant sites are located at single energy levels and arise from the intentional introduction of impurities. These states are illustrated pictorially by Figure 2-2.Acceptor Energy LevelDonor Energy LevelE cE vN(E)E N E R G Y Figure 2-2. Density of states plot representing discrete localized dopant levels. The donor levels are located positively down from the conduction band and the acceptor levels are located positively up from the valence band.The charge arising from a set of i of these discrete dopant states can be expressed asN d D + = i 2N d D,i f D,i (2.1.2.1c)if they are donor-like and from a set of j of these discrete dopant states asN d A - = i 2N d A,j f A,j (2.1.2.1d)if they are acceptor-like. Here N d D + and N d A -,represent the discrete donor and acceptor charge,respectively. We allow for a number of these levels in AMPS with volume concentrations of N d D,i and N d A,j corresponding to the donor level energy E i and the acceptor level energy E j , respectively.The number of these doping sites per volume and their energy levels may even vary with position in AMPS as specified by the user. The quantity f D,i is the probability that a discrete-level donor site of energy E i has lost an electron and f A,j is the probability that a discrete level acceptor site of energy E j has gained an electron. In thermodynamic equilibrium, the occupation probabilities f D,i and f A,j are represented by one minus the Fermi function and by the Fermi function, respectively.That is, in thermodynamic equilibriumf D,i = 11+exp !#$&E F - E i kT (2.1.2.1e)。
An Overview of Recent Progress in the Study of Distributed Multi-agent Coordination
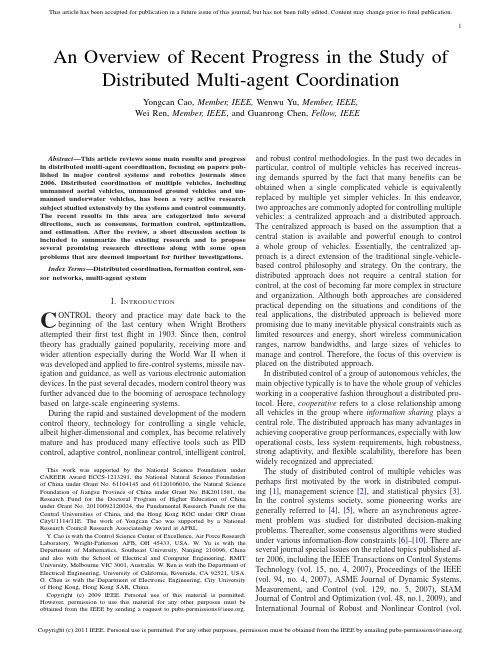
An Overview of Recent Progress in the Study of Distributed Multi-agent CoordinationYongcan Cao,Member,IEEE,Wenwu Yu,Member,IEEE,Wei Ren,Member,IEEE,and Guanrong Chen,Fellow,IEEEAbstract—This article reviews some main results and progress in distributed multi-agent coordination,focusing on papers pub-lished in major control systems and robotics journals since 2006.Distributed coordination of multiple vehicles,including unmanned aerial vehicles,unmanned ground vehicles and un-manned underwater vehicles,has been a very active research subject studied extensively by the systems and control community. The recent results in this area are categorized into several directions,such as consensus,formation control,optimization, and estimation.After the review,a short discussion section is included to summarize the existing research and to propose several promising research directions along with some open problems that are deemed important for further investigations.Index Terms—Distributed coordination,formation control,sen-sor networks,multi-agent systemI.I NTRODUCTIONC ONTROL theory and practice may date back to thebeginning of the last century when Wright Brothers attempted theirfirst testflight in1903.Since then,control theory has gradually gained popularity,receiving more and wider attention especially during the World War II when it was developed and applied tofire-control systems,missile nav-igation and guidance,as well as various electronic automation devices.In the past several decades,modern control theory was further advanced due to the booming of aerospace technology based on large-scale engineering systems.During the rapid and sustained development of the modern control theory,technology for controlling a single vehicle, albeit higher-dimensional and complex,has become relatively mature and has produced many effective tools such as PID control,adaptive control,nonlinear control,intelligent control, This work was supported by the National Science Foundation under CAREER Award ECCS-1213291,the National Natural Science Foundation of China under Grant No.61104145and61120106010,the Natural Science Foundation of Jiangsu Province of China under Grant No.BK2011581,the Research Fund for the Doctoral Program of Higher Education of China under Grant No.20110092120024,the Fundamental Research Funds for the Central Universities of China,and the Hong Kong RGC under GRF Grant CityU1114/11E.The work of Yongcan Cao was supported by a National Research Council Research Associateship Award at AFRL.Y.Cao is with the Control Science Center of Excellence,Air Force Research Laboratory,Wright-Patterson AFB,OH45433,USA.W.Yu is with the Department of Mathematics,Southeast University,Nanjing210096,China and also with the School of Electrical and Computer Engineering,RMIT University,Melbourne VIC3001,Australia.W.Ren is with the Department of Electrical Engineering,University of California,Riverside,CA92521,USA.G.Chen is with the Department of Electronic Engineering,City University of Hong Kong,Hong Kong SAR,China.Copyright(c)2009IEEE.Personal use of this material is permitted. However,permission to use this material for any other purposes must be obtained from the IEEE by sending a request to pubs-permissions@.and robust control methodologies.In the past two decades in particular,control of multiple vehicles has received increas-ing demands spurred by the fact that many benefits can be obtained when a single complicated vehicle is equivalently replaced by multiple yet simpler vehicles.In this endeavor, two approaches are commonly adopted for controlling multiple vehicles:a centralized approach and a distributed approach. The centralized approach is based on the assumption that a central station is available and powerful enough to control a whole group of vehicles.Essentially,the centralized ap-proach is a direct extension of the traditional single-vehicle-based control philosophy and strategy.On the contrary,the distributed approach does not require a central station for control,at the cost of becoming far more complex in structure and organization.Although both approaches are considered practical depending on the situations and conditions of the real applications,the distributed approach is believed more promising due to many inevitable physical constraints such as limited resources and energy,short wireless communication ranges,narrow bandwidths,and large sizes of vehicles to manage and control.Therefore,the focus of this overview is placed on the distributed approach.In distributed control of a group of autonomous vehicles,the main objective typically is to have the whole group of vehicles working in a cooperative fashion throughout a distributed pro-tocol.Here,cooperative refers to a close relationship among all vehicles in the group where information sharing plays a central role.The distributed approach has many advantages in achieving cooperative group performances,especially with low operational costs,less system requirements,high robustness, strong adaptivity,andflexible scalability,therefore has been widely recognized and appreciated.The study of distributed control of multiple vehicles was perhapsfirst motivated by the work in distributed comput-ing[1],management science[2],and statistical physics[3]. In the control systems society,some pioneering works are generally referred to[4],[5],where an asynchronous agree-ment problem was studied for distributed decision-making problems.Thereafter,some consensus algorithms were studied under various information-flow constraints[6]–[10].There are several journal special issues on the related topics published af-ter2006,including the IEEE Transactions on Control Systems Technology(vol.15,no.4,2007),Proceedings of the IEEE (vol.94,no.4,2007),ASME Journal of Dynamic Systems, Measurement,and Control(vol.129,no.5,2007),SIAM Journal of Control and Optimization(vol.48,no.1,2009),and International Journal of Robust and Nonlinear Control(vol.21,no.12,2011).In addition,there are some recent reviewsand progress reports given in the surveys[11]–[15]and thebooks[16]–[23],among others.This article reviews some main results and recent progressin distributed multi-agent coordination,published in majorcontrol systems and robotics journals since2006.Due to space limitations,we refer the readers to[24]for a more completeversion of the same overview.For results before2006,thereaders are referred to[11]–[14].Specifically,this article reviews the recent research resultsin the following directions,which are not independent but actually may have overlapping to some extent:1.Consensus and the like(synchronization,rendezvous).Consensus refers to the group behavior that all theagents asymptotically reach a certain common agreementthrough a local distributed protocol,with or without predefined common speed and orientation.2.Distributed formation and the like(flocking).Distributedformation refers to the group behavior that all the agents form a pre-designed geometrical configuration throughlocal interactions with or without a common reference.3.Distributed optimization.This refers to algorithmic devel-opments for the analysis and optimization of large-scaledistributed systems.4.Distributed estimation and control.This refers to dis-tributed control design based on local estimation aboutthe needed global information.The rest of this article is organized as follows.In Section II,basic notations of graph theory and stochastic matrices are introduced.Sections III,IV,V,and VI describe the recentresearch results and progress in consensus,formation control, optimization,and estimation.Finally,the article is concludedby a short section of discussions with future perspectives.II.P RELIMINARIESA.Graph TheoryFor a system of n connected agents,its network topology can be modeled as a directed graph denoted by G=(V,W),where V={v1,v2,···,v n}and W⊆V×V are,respectively, the set of agents and the set of edges which directionallyconnect the agents together.Specifically,the directed edgedenoted by an ordered pair(v i,v j)means that agent j can access the state information of agent i.Accordingly,agent i is a neighbor of agent j.A directed path is a sequence of directed edges in the form of(v1,v2),(v2,v3),···,with all v i∈V.A directed graph has a directed spanning tree if there exists at least one agent that has a directed path to every other agent.The union of a set of directed graphs with the same setof agents,{G i1,···,G im},is a directed graph with the sameset of agents and its set of edges is given by the union of the edge sets of all the directed graphs G ij,j=1,···,m.A complete directed graph is a directed graph in which each pair of distinct agents is bidirectionally connected by an edge,thus there is a directed path from any agent to any other agent in the network.Two matrices are used to represent the network topology: the adjacency matrix A=[a ij]∈R n×n with a ij>0if (v j,v i)∈W and a ij=0otherwise,and the Laplacian matrix L=[ℓij]∈R n×n withℓii= n j=1a ij andℓij=−a ij,i=j, which is generally asymmetric for directed graphs.B.Stochastic MatricesA nonnegative square matrix is called(row)stochastic matrix if its every row is summed up to one.The product of two stochastic matrices is still a stochastic matrix.A row stochastic matrix P∈R n×n is called indecomposable and aperiodic if lim k→∞P k=1y T for some y∈R n[25],where 1is a vector with all elements being1.III.C ONSENSUSConsider a group of n agents,each with single-integrator kinematics described by˙x i(t)=u i(t),i=1,···,n,(1) where x i(t)and u i(t)are,respectively,the state and the control input of the i th agent.A typical consensus control algorithm is designed asu i(t)=nj=1a ij(t)[x j(t)−x i(t)],(2)where a ij(t)is the(i,j)th entry of the corresponding ad-jacency matrix at time t.The main idea behind(2)is that each agent moves towards the weighted average of the states of its neighbors.Given the switching network pattern due to the continuous motions of the dynamic agents,coupling coefficients a ij(t)in(2),hence the graph topologies,are generally time-varying.It is shown in[9],[10]that consensus is achieved if the underlying directed graph has a directed spanning tree in some jointly fashion in terms of a union of its time-varying graph topologies.The idea behind consensus serves as a fundamental principle for the design of distributed multi-agent coordination algo-rithms.Therefore,investigating consensus has been a main research direction in the study of distributed multi-agent co-ordination.To bridge the gap between the study of consensus algorithms and many physical properties inherited in practical systems,it is necessary and meaningful to study consensus by considering many practical factors,such as actuation,control, communication,computation,and vehicle dynamics,which characterize some important features of practical systems.This is the main motivation to study consensus.In the following part of the section,an overview of the research progress in the study of consensus is given,regarding stochastic network topologies and dynamics,complex dynamical systems,delay effects,and quantization,mainly after2006.Several milestone results prior to2006can be found in[2],[4]–[6],[8]–[10], [26].A.Stochastic Network Topologies and DynamicsIn multi-agent systems,the network topology among all vehicles plays a crucial role in determining consensus.The objective here is to explicitly identify necessary and/or suffi-cient conditions on the network topology such that consensus can be achieved under properly designed algorithms.It is often reasonable to consider the case when the network topology is deterministic under ideal communication chan-nels.Accordingly,main research on the consensus problem was conducted under a deterministicfixed/switching network topology.That is,the adjacency matrix A(t)is deterministic. Some other times,when considering random communication failures,random packet drops,and communication channel instabilities inherited in physical communication channels,it is necessary and important to study consensus problem in the stochastic setting where a network topology evolves according to some random distributions.That is,the adjacency matrix A(t)is stochastically evolving.In the deterministic setting,consensus is said to be achieved if all agents eventually reach agreement on a common state. In the stochastic setting,consensus is said to be achieved almost surely(respectively,in mean-square or in probability)if all agents reach agreement on a common state almost surely (respectively,in mean-square or with probability one).Note that the problem studied in the stochastic setting is slightly different from that studied in the deterministic setting due to the different assumptions in terms of the network topology. Consensus over a stochastic network topology was perhaps first studied in[27],where some sufficient conditions on the network topology were given to guarantee consensus with probability one for systems with single-integrator kinemat-ics(1),where the rate of convergence was also studied.Further results for consensus under a stochastic network topology were reported in[28]–[30],where research effort was conducted for systems with single-integrator kinematics[28],[29]or double-integrator dynamics[30].Consensus for single-integrator kine-matics under stochastic network topology has been exten-sively studied in particular,where some general conditions for almost-surely consensus was derived[29].Loosely speaking, almost-surely consensus for single-integrator kinematics can be achieved,i.e.,x i(t)−x j(t)→0almost surely,if and only if the expectation of the network topology,namely,the network topology associated with expectation E[A(t)],has a directed spanning tree.It is worth noting that the conditions are analogous to that in[9],[10],but in the stochastic setting. In view of the special structure of the closed-loop systems concerning consensus for single-integrator kinematics,basic properties of the stochastic matrices play a crucial role in the convergence analysis of the associated control algorithms. Consensus for double-integrator dynamics was studied in[30], where the switching network topology is assumed to be driven by a Bernoulli process,and it was shown that consensus can be achieved if the union of all the graphs has a directed spanning tree.Apparently,the requirement on the network topology for double-integrator dynamics is a special case of that for single-integrator kinematics due to the difference nature of thefinal states(constantfinal states for single-integrator kinematics and possible dynamicfinal states for double-integrator dynamics) caused by the substantial dynamical difference.It is still an open question as if some general conditions(corresponding to some specific algorithms)can be found for consensus with double-integrator dynamics.In addition to analyzing the conditions on the network topology such that consensus can be achieved,a special type of consensus algorithm,the so-called gossip algorithm[31],[32], has been used to achieve consensus in the stochastic setting. The gossip algorithm can always guarantee consensus almost surely if the available pairwise communication channels satisfy certain conditions(such as a connected graph).The way of network topology switching does not play any role in the consideration of consensus.The current study on consensus over stochastic network topologies has shown some interesting results regarding:(1) consensus algorithm design for various multi-agent systems,(2)conditions of the network topologies on consensus,and(3)effects of the stochastic network topologies on the con-vergence rate.Future research on this topic includes,but not limited to,the following two directions:(1)when the network topology itself is stochastic,how to determine the probability of reaching consensus almost surely?(2)compared with the deterministic network topology,what are the advantages and disadvantages of the stochastic network topology,regarding such as robustness and convergence rate?As is well known,disturbances and uncertainties often exist in networked systems,for example,channel noise,commu-nication noise,uncertainties in network parameters,etc.In addition to the stochastic network topologies discussed above, the effect of stochastic disturbances[33],[34]and uncertain-ties[35]on the consensus problem also needs investigation. Study has been mainly devoted to analyzing the performance of consensus algorithms subject to disturbances and to present-ing conditions on the uncertainties such that consensus can be achieved.In addition,another interesting direction in dealing with disturbances and uncertainties is to design distributed localfiltering algorithms so as to save energy and improve computational efficiency.Distributed localfiltering algorithms play an important role and are more effective than traditional centralizedfiltering algorithms for multi-agent systems.For example,in[36]–[38]some distributed Kalmanfilters are designed to implement data fusion.In[39],by analyzing consensus and pinning control in synchronization of complex networks,distributed consensusfiltering in sensor networks is addressed.Recently,Kalmanfiltering over a packet-dropping network is designed through a probabilistic approach[40]. Today,it remains a challenging problem to incorporate both dynamics of consensus and probabilistic(Kalman)filtering into a unified framework.plex Dynamical SystemsSince consensus is concerned with the behavior of a group of vehicles,it is natural to consider the system dynamics for practical vehicles in the study of the consensus problem. Although the study of consensus under various system dynam-ics is due to the existence of complex dynamics in practical systems,it is also interesting to observe that system dynamics play an important role in determining thefinal consensus state.For instance,the well-studied consensus of multi-agent systems with single-integrator kinematics often converges to a constantfinal value instead.However,consensus for double-integrator dynamics might admit a dynamicfinal value(i.e.,a time function).These important issues motivate the study of consensus under various system dynamics.As a direct extension of the study of the consensus prob-lem for systems with simple dynamics,for example,with single-integrator kinematics or double-integrator dynamics, consensus with general linear dynamics was also studied recently[41]–[43],where research is mainly devoted tofinding feedback control laws such that consensus(in terms of the output states)can be achieved for general linear systems˙x i=Ax i+Bu i,y i=Cx i,(3) where A,B,and C are constant matrices with compatible sizes.Apparently,the well-studied single-integrator kinematics and double-integrator dynamics are special cases of(3)for properly choosing A,B,and C.As a further extension,consensus for complex systems has also been extensively studied.Here,the term consensus for complex systems is used for the study of consensus problem when the system dynamics are nonlinear[44]–[48]or with nonlinear consensus algorithms[49],[50].Examples of the nonlinear system dynamics include:•Nonlinear oscillators[45].The dynamics are often as-sumed to be governed by the Kuramoto equation˙θi=ωi+Kstability.A well-studied consensus algorithm for(1)is given in(2),where it is now assumed that time delay exists.Two types of time delays,communication delay and input delay, have been considered in the munication delay accounts for the time for transmitting information from origin to destination.More precisely,if it takes time T ij for agent i to receive information from agent j,the closed-loop system of(1)using(2)under afixed network topology becomes˙x i(t)=nj=1a ij(t)[x j(t−T ij)−x i(t)].(7)An interpretation of(7)is that at time t,agent i receives information from agent j and uses data x j(t−T ij)instead of x j(t)due to the time delay.Note that agent i can get its own information instantly,therefore,input delay can be considered as the summation of computation time and execution time. More precisely,if the input delay for agent i is given by T p i, then the closed-loop system of(1)using(2)becomes˙x i(t)=nj=1a ij(t)[x j(t−T p i)−x i(t−T p i)].(8)Clearly,(7)refers to the case when only communication delay is considered while(8)refers to the case when only input delay is considered.It should be emphasized that both communication delay and input delay might be time-varying and they might co-exist at the same time.In addition to time delay,it is also important to consider packet drops in exchanging state information.Fortunately, consensus with packet drops can be considered as a special case of consensus with time delay,because re-sending packets after they were dropped can be easily done but just having time delay in the data transmission channels.Thus,the main problem involved in consensus with time delay is to study the effects of time delay on the convergence and performance of consensus,referred to as consensusabil-ity[52].Because time delay might affect the system stability,it is important to study under what conditions consensus can still be guaranteed even if time delay exists.In other words,can onefind conditions on the time delay such that consensus can be achieved?For this purpose,the effect of time delay on the consensusability of(1)using(2)was investigated.When there exists only(constant)input delay,a sufficient condition on the time delay to guarantee consensus under afixed undirected interaction graph is presented in[8].Specifically,an upper bound for the time delay is derived under which consensus can be achieved.This is a well-expected result because time delay normally degrades the system performance gradually but will not destroy the system stability unless the time delay is above a certain threshold.Further studies can be found in, e.g.,[53],[54],which demonstrate that for(1)using(2),the communication delay does not affect the consensusability but the input delay does.In a similar manner,consensus with time delay was studied for systems with different dynamics, where the dynamics(1)are replaced by other more complex ones,such as double-integrator dynamics[55],[56],complex networks[57],[58],rigid bodies[59],[60],and general nonlinear dynamics[61].In summary,the existing study of consensus with time delay mainly focuses on analyzing the stability of consensus algo-rithms with time delay for various types of system dynamics, including linear and nonlinear dynamics.Generally speaking, consensus with time delay for systems with nonlinear dynam-ics is more challenging.For most consensus algorithms with time delays,the main research question is to determine an upper bound of the time delay under which time delay does not affect the consensusability.For communication delay,it is possible to achieve consensus under a relatively large time delay threshold.A notable phenomenon in this case is that thefinal consensus state is constant.Considering both linear and nonlinear system dynamics in consensus,the main tools for stability analysis of the closed-loop systems include matrix theory[53],Lyapunov functions[57],frequency-domain ap-proach[54],passivity[58],and the contraction principle[62]. Although consensus with time delay has been studied extensively,it is often assumed that time delay is either constant or random.However,time delay itself might obey its own dynamics,which possibly depend on the communication distance,total computation load and computation capability, etc.Therefore,it is more suitable to represent the time delay as another system variable to be considered in the study of the consensus problem.In addition,it is also important to consider time delay and other physical constraints simultaneously in the study of the consensus problem.D.QuantizationQuantized consensus has been studied recently with motiva-tion from digital signal processing.Here,quantized consensus refers to consensus when the measurements are digital rather than analog therefore the information received by each agent is not continuous and might have been truncated due to digital finite precision constraints.Roughly speaking,for an analog signal s,a typical quantizer with an accuracy parameterδ, also referred to as quantization step size,is described by Q(s)=q(s,δ),where Q(s)is the quantized signal and q(·,·) is the associated quantization function.For instance[63],a quantizer rounding a signal s to its nearest integer can be expressed as Q(s)=n,if s∈[(n−1/2)δ,(n+1/2)δ],n∈Z, where Z denotes the integer set.Note that the types of quantizers might be different for different systems,hence Q(s) may differ for different systems.Due to the truncation of the signals received,consensus is now considered achieved if the maximal state difference is not larger than the accuracy level associated with the whole system.A notable feature for consensus with quantization is that the time to reach consensus is usuallyfinite.That is,it often takes afinite period of time for all agents’states to converge to an accuracy interval.Accordingly,the main research is to investigate the convergence time associated with the proposed consensus algorithm.Quantized consensus was probablyfirst studied in[63], where a quantized gossip algorithm was proposed and its convergence was analyzed.In particular,the bound of theconvergence time for a complete graph was shown to be poly-nomial in the network size.In[64],coding/decoding strate-gies were introduced to the quantized consensus algorithms, where it was shown that the convergence rate depends on the accuracy of the quantization but not the coding/decoding schemes.In[65],quantized consensus was studied via the gossip algorithm,with both lower and upper bounds of the expected convergence time in the worst case derived in terms of the principle submatrices of the Laplacian matrix.Further results regarding quantized consensus were reported in[66]–[68],where the main research was also on the convergence time for various proposed quantized consensus algorithms as well as the quantization effects on the convergence time.It is intuitively reasonable that the convergence time depends on both the quantization level and the network topology.It is then natural to ask if and how the quantization methods affect the convergence time.This is an important measure of the robustness of a quantized consensus algorithm(with respect to the quantization method).Note that it is interesting but also more challenging to study consensus for general linear/nonlinear systems with quantiza-tion.Because the difference between the truncated signal and the original signal is bounded,consensus with quantization can be considered as a special case of one without quantization when there exist bounded disturbances.Therefore,if consensus can be achieved for a group of vehicles in the absence of quantization,it might be intuitively correct to say that the differences among the states of all vehicles will be bounded if the quantization precision is small enough.However,it is still an open question to rigorously describe the quantization effects on consensus with general linear/nonlinear systems.E.RemarksIn summary,the existing research on the consensus problem has covered a number of physical properties for practical systems and control performance analysis.However,the study of the consensus problem covering multiple physical properties and/or control performance analysis has been largely ignored. In other words,two or more problems discussed in the above subsections might need to be taken into consideration simul-taneously when studying the consensus problem.In addition, consensus algorithms normally guarantee the agreement of a team of agents on some common states without taking group formation into consideration.To reflect many practical applications where a group of agents are normally required to form some preferred geometric structure,it is desirable to consider a task-oriented formation control problem for a group of mobile agents,which motivates the study of formation control presented in the next section.IV.F ORMATION C ONTROLCompared with the consensus problem where thefinal states of all agents typically reach a singleton,thefinal states of all agents can be more diversified under the formation control scenario.Indeed,formation control is more desirable in many practical applications such as formationflying,co-operative transportation,sensor networks,as well as combat intelligence,surveillance,and reconnaissance.In addition,theperformance of a team of agents working cooperatively oftenexceeds the simple integration of the performances of all individual agents.For its broad applications and advantages,formation control has been a very active research subject inthe control systems community,where a certain geometric pattern is aimed to form with or without a group reference.More precisely,the main objective of formation control is to coordinate a group of agents such that they can achievesome desired formation so that some tasks can befinished bythe collaboration of the agents.Generally speaking,formation control can be categorized according to the group reference.Formation control without a group reference,called formationproducing,refers to the algorithm design for a group of agents to reach some pre-desired geometric pattern in the absenceof a group reference,which can also be considered as the control objective.Formation control with a group reference,called formation tracking,refers to the same task but followingthe predesignated group reference.Due to the existence of the group reference,formation tracking is usually much morechallenging than formation producing and control algorithmsfor the latter might not be useful for the former.As of today, there are still many open questions in solving the formationtracking problem.The following part of the section reviews and discussesrecent research results and progress in formation control, including formation producing and formation tracking,mainlyaccomplished after2006.Several milestone results prior to 2006can be found in[69]–[71].A.Formation ProducingThe existing work in formation control aims at analyzingthe formation behavior under certain control laws,along with stability analysis.1)Matrix Theory Approach:Due to the nature of multi-agent systems,matrix theory has been frequently used in thestability analysis of their distributed coordination.Note that consensus input to each agent(see e.g.,(2))isessentially a weighted average of the differences between the states of the agent’s neighbors and its own.As an extensionof the consensus algorithms,some coupling matrices wereintroduced here to offset the corresponding control inputs by some angles[72],[73].For example,given(1),the controlinput(2)is revised as u i(t)= n j=1a ij(t)C[x j(t)−x i(t)], where C is a coupling matrix with compatible size.If x i∈R3, then C can be viewed as the3-D rotational matrix.The mainidea behind the revised algorithm is that the original controlinput for reaching consensus is now rotated by some angles. The closed-loop system can be expressed in a vector form, whose stability can be determined by studying the distribution of the eigenvalues of a certain transfer matrix.Main research work was conducted in[72],[73]to analyze the collective motions for systems with single-integrator kinematics and double-integrator dynamics,where the network topology,the damping gain,and C were shown to affect the collective motions.Analogously,the collective motions for a team of nonlinear self-propelling agents were shown to be affected by。
Inhomogeneous d-wave superconducting state of a doped Mott insulator
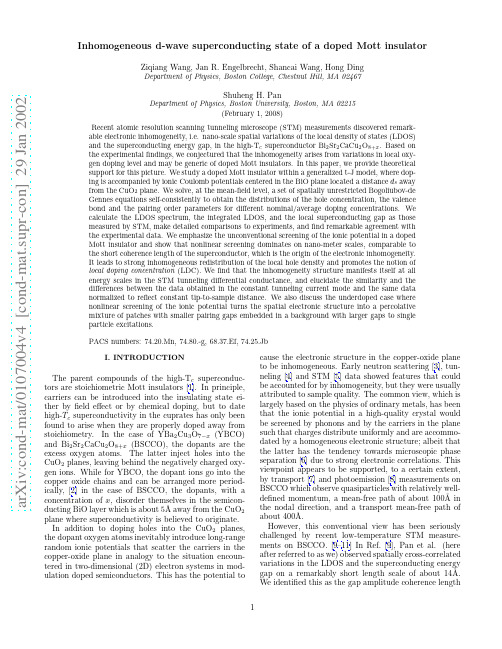
cause the electronic structure in the copper-oxide plane to be inhomogeneous. Early neutron scattering [3], tunneling [4] and STM [5] data showed features that could be accounted for by inhomogeneity, but they were usually attributed to sample quality. The common view, which is largely based on the physics of ordinary metals, has been that the ionic potential in a high-quality crystal would be screened by phonons and by the carriers in the plane such that charges distribute uniformly and are accommodated by a homogeneous electronic structure; albeit that the latter has the tendency towards microscopic phase separation [6] due to strong electronic correlations. This viewpoint appears to be supported, to a certain extent, by transport [7] and photoemission [8] measurements on BSCCO which observe quasiparticles with relatively welldefined momentum, a mean-free path of about 100˚ A in the nodal direction, and a transport mean-free path of about 400˚ A. However, this conventional view has been seriously challenged by recent low-temperature STM measurements on BSCCO. [9–11] In Ref. [9], Pan et al. (here after referred to as we) observed spatially cross-correlated variations in the LDOS and the superconducting energy gap on a remarkably short length scale of about 14˚ A. We identified this as the gap amplitude coherence length 1
Graphics Hardware (2004) T. Akenine-Mller, M. McCool (Editors) Efficient Partitioning of Fr
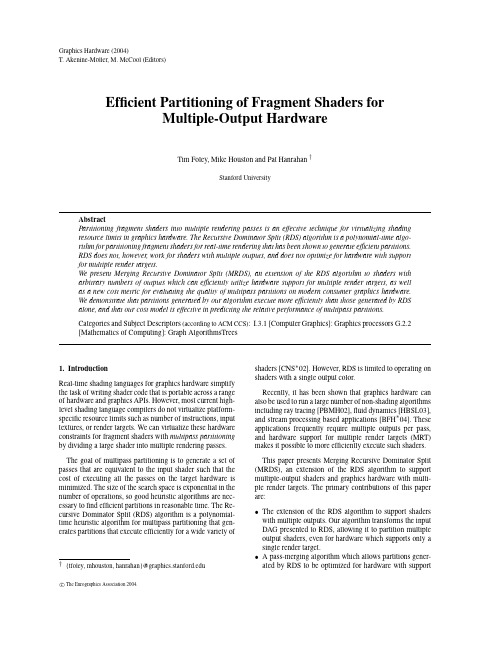
Graphics Hardware(2004)T.Akenine-Möller,M.McCool(Editors)Efficient Partitioning of Fragment Shaders forMultiple-Output HardwareTim Foley,Mike Houston and Pat Hanrahan†Stanford UniversityAbstractPartitioning fragment shaders into multiple rendering passes is an effective technique for virtualizing shading resource limits in graphics hardware.The Recursive Dominator Split(RDS)algorithm is a polynomial-time algo-rithm for partitioning fragment shaders for real-time rendering that has been shown to generate efficient partitions.RDS does not,however,work for shaders with multiple outputs,and does not optimize for hardware with support for multiple render targets.We present Merging Recursive Dominator Split(MRDS),an extension of the RDS algorithm to shaders with arbitrary numbers of outputs which can efficiently utilize hardware support for multiple render targets,as well as a new cost metric for evaluating the quality of multipass partitions on modern consumer graphics hardware.We demonstrate that partitions generated by our algorithm execute more efficiently than those generated by RDS alone,and that our cost model is effective in predicting the relative performance of multipass partitions.Categories and Subject Descriptors(according to ACM CCS):I.3.1[Computer Graphics]:Graphics processors G.2.2 [Mathematics of Computing]:Graph AlgorithmsTrees1.IntroductionReal-time shading languages for graphics hardware simplify the task of writing shader code that is portable across a range of hardware and graphics APIs.However,most current high-level shading language compilers do not virtualize platform-specific resource limits such as number of instructions,input textures,or render targets.We can virtualize these hardware constraints for fragment shaders with multipass partitioning by dividing a large shader into multiple rendering passes. The goal of multipass partitioning is to generate a set of passes that are equivalent to the input shader such that the cost of executing all the passes on the target hardware is minimized.The size of the search space is exponential in the number of operations,so good heuristic algorithms are nec-essary tofind efficient partitions in reasonable time.The Re-cursive Dominator Split(RDS)algorithm is a polynomial-time heuristic algorithm for multipass partitioning that gen-erates partitions that execute efficiently for a wide variety of †{tfoley,mhouston,hanrahan}@ shaders[CNS∗02].However,RDS is limited to operating on shaders with a single output color.Recently,it has been shown that graphics hardware can also be used to run a large number of non-shading algorithms including ray tracing[PBMH02],fluid dynamics[HBSL03], and stream processing based applications[BFH∗04].These applications frequently require multiple outputs per pass, and hardware support for multiple render targets(MRT) makes it possible to more efficiently execute such shaders. This paper presents Merging Recursive Dominator Split (MRDS),an extension of the RDS algorithm to support multiple-output shaders and graphics hardware with multi-ple render targets.The primary contributions of this paper are:•The extension of the RDS algorithm to support shaders with multiple outputs.Our algorithm transforms the input DAG presented to RDS,allowing it to partition multiple output shaders,even for hardware which supports only a single render target.•A pass-merging algorithm which allows partitions gener-ated by RDS to be optimized for hardware with supportc The Eurographics Association2004.for multiple render targets.We derive two new algorithms for multipass partitioning,MRDS and MRDS ,that com-bine merging with RDS.•Performance analysis demonstrating that MRDS produces more efficient partitions of fragment shaders than RDS. We show that even for shaders with only a single output value,partitions generated by MRDS can execute more efficiently on graphics hardware with MRT support. 2.Related Work2.1.High-Level Shading LanguagesCook[Coo84]and Perlin[Per85]laid the groundwork for current shading languages.The Renderman shading lan-guage[HL90]is commonly used today for high-quality of-fline shading in software rendering systems.The Pixelflow shading system introduced pfman,a shading language for real-time rendering[OL98].The Stanford Real-Time Shading Language(RTSL)sys-tem compiles a Renderman-like language for early pro-grammable graphics hardware[PMTH01].RTSL virtual-izes“frequencies”of computation,allowing the compiler to select whether individual operations will be executed per-vertex or per-fragment.NVIDIA’s Cg[MGAK03]and Microsoft’s HLSL[Mic03] are high-level languages for current graphics hardware that allow programs to be written for either the vertex or frag-ment processor and then compiled for a variety of hardware targets.While these languages do not encode anyfixed re-source limitations,the individual compiler targets have strict limits and will reject shaders that exceed them.Thus,pro-grammers must write shader implementations for multiple hardware targets,manually breaking shaders that exceed re-source limits into multiple passes.The Sh system is a meta-compiler implemented in C++ for generating shaders at runtime and applying transforma-tions to them[MQP02,MMT04].The system does not ex-plicitly specify resource limitations,although large shaders can currently fail to execute on graphics hardware.The OpenGL Shading Language,GLSL,is a high-level languages for writing vertex and fragment processor shaders that makes it easy for programmable shaders to integrate with state from thefixed-function pipeline[KBR03].The GLSL specification requires that implementations virtualize limits on number of instructions and temporary registers,but forces programmers to query and respect hardware-specific limitations on other resources.2.2.Multipass PartitioningPeercy,et al.map a subset of the Renderman language to a fixed-function OpenGL platform by abstracting the graphics hardware as a SIMD processor[POAU00].Each rendering pass executes a single SIMD instruction,and a tree-matching approach is used to map shader computations to the small set offixed-function operations available.While this technique generates good multipass partitions forfixed-function hard-ware,Proudfoot et al.demonstrate that tree-matching tech-niques are not sufficient for multipass partitioning on pro-grammable hardware[PMTH01].RTSL utilizes the RDS algorithm to virtualize fragment shading resource limits through multipass partitioning.As shown in[CNS∗02],this allows for efficient partitioning of large shaders into multiple passes.The Ashli system reads shaders in a number of high-level languages as input,including HLSL,GLSL,and Render-man,and generates low-level code to execute them on graph-ics hardware[ATI03a].Ashli can generate multipass par-titions for large shaders using RDS and provides the user with API calls to progressively render their scene.Ashli has demonstrated the effectiveness of RDS with multiple input languages.Both the RTSL and Ashli systems are able to load bal-ance shading computations between the vertex and fragment processors.Our implementation does not consider splitting shaders across the two shading units,only concentrating on partitioning shaders to run on the fragment processor.2.3.Overview of the RDS AlgorithmGiven a fragment shader represented as a DAG,the prob-lem of generating a multipass partition is as follows:label a subset of the n DAG nodes as splits,intermediate values to be saved to texture memory,and then generate a partition of those splits into p rendering passes.Each pass then consists of shader code to generate its constituent splits as outputs, using texture fetches to restore the values of previously com-puted splits.Such a partition is valid if each of the passes can run on the target hardware and they can be ordered to pre-serve dependencies between splits.Among the many possi-ble multipass partitions of a given shader,we wish tofind one that is maximally efficient.However,the space of pos-sible partitions is exponential,so the goal of multipass par-titioning is to efficientlyfind a partition that is close to opti-mal.The RDS algorithm combines top-down heuristic split-ting,bottom-up greedy merging,and a limited search algo-rithm to mark nodes in a shader DAG as splits.We use the RDS algorithm as the base for our new techniques.RDS as-sumes that the target hardware can only output a single value per fragment in each rendering pass,and thus that the shader DAG has a single root.Along with this graph,RDS operates on its associated dominator tree.A DAG node x is a domi-nator of node y,written x dom y,if all paths from the root of the graph to y pass through x.The immediate dominator of node y is the unique x=y such that x dom y and for all nodes z=y,z dom y⇒z dom x.The dominator tree of ac The Eurographics Association2004.DAG shares the node set of the graph,and connects eachnode as the direct child of its immediate dominator. Some subset of the nodes in the DAG are multiply-referenced(MR)nodes,having more than one direct par-ent.The RDS algorithm is primarily concerned with whether these MR nodes should be marked as split locations.If a MR node is marked as a split,we subsequently incur the addi-tional bandwidth costs of writing the value to texture mem-ory and restoring that value in later passes.If the node is not marked as a split,we may incur additional computation costs from recomputing its value in multiple passes.Both of these additional costs can be eliminated if we can compute a MR node in the same pass as its immediate dominator,as all ref-erences to the MR node are then isolated to a single pass.The RDS algorithm attempts to eliminate save/recompute costs when possible and otherwise tries to chose the less expen-sive of the two options.RDS uses two graph traversals to partition the DAG,a top-down subdivision over the dominator tree,and a bottom-up merge over the DAG.The subdivision traversal makes heuristic decisions about whether to save or recompute MR nodes,while the merge traversal greedily combines nodes with as many of their children as possible.These two traver-sals operate over the n nodes of the DAG and at each node use a low-level compiler to check the validity of afixed number of subregions of the graph.Assuming that low-level compilation is a linear-time operation,this leads to an over-all running time of O(n2).The graph traversals are wrapped in a limited search over the MR nodes of the DAG.The search algorithm forces successive MR nodes to be saved or recomputed(split,or unsplit)and compares the cost of partitions generated by the graph traversals under these two constraints.Each node is thenfixed in whatever state led to the better partition and this information overrides the heuris-tic decisions made in the subdivide ing this limited search can increase the efficiency of generated partitions,but increases running times by a factor of n,making RDS an O(n3)algorithm.3.The Algorithm3.1.Multiple-Output ShadersThe RDS algorithm cannot operate on shaders with multiple output values since the dominator tree which drives the sub-division step is undefined when the shader DAG has multiple roots.A MR node m that can be reached from two different DAG roots(outputs)may have no immediate dominator,and thus would not be considered by the subdivide traversal of RDS.We propose a simple solution to this problem that still al-lows us to take advantage of the information the dominator tree provides.Before applying RDS to a shader DAG with multiple root nodes,we insert a new node at the root of the DAG with operation join and having the shaders outputsas(a)(b)Shader DAG Dominator GraphFigure1:(a)A multiple-output shader and its associated dominator graph.Note that the shaded intermediate nodes do not have immediate dominators,and the dominator graph is not a tree.(b)After adding a new root node to the DAG that joins the two outputs,the dominator graph is tree-structured.children.We define the join operation so that it compiles suc-cessfully if and only if all of its children are marked as splits. Figure1illustrates how this procedure is applied to a DAG with multiple roots.The left graphs represent shading DAGs and the right graphs are their associated dominator graphs. The addition of the join operation generate a single-rooted DAG from which a dominator tree can be derived.In this way we can partition multiple-output shaders into multiple single-output rendering passes while remaining transparent to the existing RDS algorithm.The result of the above algorithm will be a set of splits, and for hardware that can only write a single output per pass we can simply assign each split to its own rendering pass to achieve good results.However,on graphics hardware that supports multiple render targets,we may be able to produce more efficient partitions.3.2.Multiple-Output HardwareTypically,the outputs of multiple-output shaders will not be disjoint,and thus intermediate results may be shared be-tween outputs.The immediate dominator of such intermedi-ate value nodes is the root join operation,and thus the dom-inator tree cannot help us to eliminate the save/recompute costs for such nodes.However,if we can write all of the out-puts that depend on such a node m in the same pass that we use to compute m,we can avoid these additional costs. This situation is not unique to shaders with multiple out-puts.As Figure2demonstrates,even in shaders with only a single output,there may be sets of intermediate values that can be computed together much more efficiently than apart. We can extend the concept of dominance in a graph to de-scribe this situation:c The Eurographics Association2004....y(b)(a)(c)Figure 2:A “ladder”configuration of intermediate value nodes.(a)The nodes x and y both depend on all previous intermediate values.(b)If x and y are marked as splits by a single-output partitioning algorithm,then the shaded nodes will have to be recomputed.(c)A MRT-aware algorithm can save both splits in a single pass and avoid the recomputa-tion.Given a DAG node x and a set of nodes S ,we say that x is a dominating set of x ,written S dom x if and only if ev-ery path from a root of the DAG to node x passes through a node in S .The parents of a node x form a dominating set of x ,and the set of shader outputs dominate every node in a shader DAG.Given this notation,we can generalize some of our previous statements about how dominance affects mul-tipass partitioning:if a DAG node x is computed in a pass with output set S ,where S dom x ,then we can eliminate any save/recompute costs for x .We can realize the benefits of this effect incrementally by merging the passes of an existing multipass partition.If an MR node n is being recomputed in many passes,and two of those passes are merged then some recomputation costs for m are eliminated.By repeatedly merging passes it may be possible to merge m with a dominating set and eliminate all of these costs.The following algorithm,MERGE-PASSES,uses greedy pass-merging to decrease recomputation costs in a multipass partition://input:T a set of splits as generated by RDS MERGE-PASSES(T )//generate initial set of passes for each node n in Tcreate a pass with output n and add it to the list of passes //create list of candidate merges for each pair (x ,y )of nodes in Tscore ←TEST-MERGE(pass (x ),pass (y ))if score ≥0thenadd (score ,x ,y )to array A of potential merges sort A in decreasing order by score//attempt to execute merges from best to worst for each tuple (score ,x ,y )in A if pass (x )=pass (y )then//score may have changed,so revalidatescore ←TEST-MERGE(pass (x ),pass (y ))if score ≥0thenEXECUTE-MERGE(pass (x ),pass (y ))//input:A,B passes (sets of nodes)//output:a score measuring how “good”the merge is TEST-MERGE(A ,B )//if there is an ordering conflict,we cannot merge if ancestors (A )∩descendants (B )=∅∨descendants (A )∩ancestors (B )=∅then return −1M ←out puts (A )∪out puts (B )//use low-level compiler to check validity if pass-valid (M )then//score is how much the merge would improve partition cost cost merged ←pass-cost (M )return cost (A )+cost (B )−cost merged else return −1//input:A,B passes (sets of nodes)EXECUTE-MERGE(A ,B )//create and initialize the merged pass create pass Pout puts (P )←out puts (A )∪out puts (B )cost (P )←pass-cost (out puts (P ))remove A ,B from list of passes add P to list of passes//update membership information for nodes in this pass for each node n in out puts (P )pass (n )←PThis algorithm operates on a list of splits and produces a list of passes.It begins by considering all possible pair-wise merges between splits and determining whether they are valid.If there exists some split z such that z is an ances-tor of one pass and a descendant of the other (i.e.z depends on the outputs of A and an output of B depends on z )we dis-miss the merge as invalid.Otherwise we construct set M ,the union of the output splits of passes A and B .If the elements of M cannot be generated in a single rendering pass on the target hardware,then the merge is invalid.For every valid merge we calculate a score measuring the improvement in cost of the merged pass over the two input passes,dismiss-ing merges that yield negative scores.We iterate over the remaining potential merges in order of decreasing score and try to execute them.It is possible that earlier merges will have invalidated a potential merge or that it will no longer improve the overall score,so we re-check validity before ex-ecuting any given merge.The running time of this algorithm is dominated by the initial search for valid merges.For an n -node DAG and s splits,the search operates over s 2pairs of splits and per-forms size-s set operations and an O (n )compiler call.This yields an overall running time of O (s 2(s +n ))for MERGE-PASSES.In general,s is O (n )in the number of DAG nodes,and thus the algorithm is O (n 3),although in practice we ex-pect s to be small relative to n .cThe Eurographics Association 2004.If the MERGE-PASSES algorithm is run directly on the results of our multiple-output enabled RDS,then the result is an O(n3)algorithm for generating multipass partitions for MRT hardware.We call this algorithm MRDS,or Merging Recursive Dominator Split.We will demonstrate in the fol-lowing section that MRDS can significantly improve the ef-ficiency of multipass partitions generated by RDS. However,it is possible that MRDS might produce poor partitions for certain shaders.Sets of splits that lead to ex-pensive partitions when executed with one split per pass might yield highly optimized partitions after greedy merg-ing.The search algorithm employed by RDS does not con-sider the potential for merging when evaluating the rela-tive quality of partitions,and thus could make sub-optimal save/recompute decisions based on the incomplete informa-tion available to it.Thus,we introduce a second modified RDS algorithm,MRDS .The MRDS algorithm integrates greedy pass-merging di-rectly into the search performed by RDS.When RDS evalu-ates the cost of a partition in order to make save/recompute decisions,wefirst apply pass-merging to collapse the candi-date set of splits into a smaller number of passes.While this modification provides the RDS search with more accurate cost information,it comes with a penalty in asymptotic per-formance.The RDS algorithm wraps a linear search around an O(n2)graph traversal,and MRDS introduces the addi-tional cost of O(n3)merging inside this search.Thus MRDS increases compile times by a factor of n over RDS,resulting in an O(n4)algorithm.3.3.ImplementationWe have implemented the RDS,MRDS and MRDS al-gorithms for multipass partitioning into the BrookGPU [BFH∗04]system for stream computing on GPUs.Although the Brook language and the BrookGPU system are de-signed for stream computation rather than interactive shad-ing,the implementation of our multipass partitioning al-gorithms are devoid of any concepts specific to streaming. The BrookGPU compiler transforms functions written in the Brook language into high-level fragment shaders,construct-ing a shader DAG from this representation.Our multipass partitioning algorithms operate on this DAG,using modu-larized compiler back-ends to encapsulate the validity test-ing and cost metrics required.In order to evaluate the ef-fectiveness of MRDS and MRDS at generating multipass partitions for hardware with support for multiple render tar-gets,we developed a back-end that generates DirectX9pixel shader code for the ATI Radeon9800XT[Mic01,ATI03b]. This back-end uses the Microsoft HLSL compiler,fxc to per-form validation and generate low-level code.Whereas the RDS implementation used in RTSL operates over a DAG of low-level machine instructions,our system operates over a DAG of the high-level expression tree and relies on the external compiler to perform instruction selec-tion.This makes implementing a back-end target much sim-pler as it need only pass high-level code to an external com-piler.However,opportunities for optimization could be lost by operating on code before instruction selection.Our im-plementation will not split shader operations that are repre-sented as primitive function calls,such as vector cross prod-uct,across passes even if they consist of multiple machine instructions.Furthermore,we generate DAG nodes for oper-ations,such as swizzles and negation,which might be free on a particular hardware target.3.4.Cost MetricAll of our multipass partitioning algorithms rely on a cost metric to provide predictions of shader performance on the target hardware.The original RDS implementation used a linear cost model that combined the total number of passes, arithmetic instructions and texture-fetch instructions in a given shader partition.In trying to model shader execution cost on our target platform,we have extended this model. Chan et al.estimate the cost of each shader pass by mea-suring the time taken to render a single-pixel quad on the target hardware and convert this into units of GPU instruc-tion cycles.The goal is to measure the cost of API overhead and setup to render a primitive,while making the cost of any per-fragment operations negligible[CNS∗02].However,be-cause shader execution is typically asynchronous with API calls on the CPU,this overhead can be mitigated by running a shader on enough data so that GPU execution time domi-nates API overhead.We expect that in practice most appli-cations of MRDS will apply to shaders being run on suffi-ciently large datasets to be GPU-limited rather than CPU-limited.Therefore we do not use the same procedure to de-rive per-pass overhead as Chan et al.However,this is not to say that we do not account for per-pass overhead.Experimentally we have found that the dom-inant overhead of each rendering pass is in writing shader results to off-chip framebuffer memory.We measured this cost by comparing execution times t0when running i in-structions over n fragments and t1when running i/2in-structions over2n fragments,always operating on enough data to be GPU-limited.If wefit a cost model of the form cost=c i·i·n+c p·n then we expect tofind c p=(t1−t0)/n. We have experimentally measured c p,the per-fragment pass overhead,to be equivalent to approximately10instructions on our target platform.We must also take into account the total number of outputs being written in a given pass,as each output incurs an addi-tional bandwidth penalty.We have found that shader output writes appear to mask the instruction execution time of small shaders.We have determined that single-output shaders with only a single instruction take the same amount of time to ex-ecute as those with6instructions,and that this number ofc The Eurographics Association2004.instructions increases linearly as we increase the number of shader outputs.We have measured the latency of a texture fetch on our target hardware to be equivalent to approximately8instruc-tions for textures infloat4format.However,accounting for this delay with a linear term fails to take into account the potential for the hardware to schedule instructions to hide the latency of texture fetches.In the best case,the pipeline can completely hide texture latency and our execution time is max(t textureFetch,t instructionExecute),while in the worst case every texture fetch leads to a stall,and execution time is t textureFetch+t instructionExecute.Rather than try to inspect the texture-access behavior of a pass,we choose to model this term as a simple average of the best-case and worst-case forms.The complete equation that we use to measure the cost c pass of a shader pass consisting of i total instructions,t tex-ture fetches and writing o output values is:c pass=c p+max(c o·o,(i+c t·t)+max(i,c t·t)) where c p=10is the per-fragment pass overhead,c o=6is the number of instructions masked by each output write,and c t=8is the latency of a texture fetch.4.ResultsIn order to evaluate the quality of partitions generated by our new algorithms,we applied four different partitioning strate-gies to four different shaders written in the Brook language. The partitioning strategies were:•Ideal-Represents a partitioning strategy that always puts all computation into a single rendering pass.The parti-tions generated by this strategy cannot be run on the target hardware,but are useful for comparison.•RDS-The original RDS algorithm modified to accept multiple-output shaders.•MRDS-The RDS strategy followed by our greedy pass-merging algorithm.•MRDS -The RDS strategy with greedy pass-merging in-tegrated into the RDS search step.The applications were selected to provide a range of shader sizes,outputs,and uses.The individual applications are:•Particle-A shader that advances a particle-system based cloth simulation.This shader uses two outputs to write the new position and velocity of each particle in the system. Each particle is constrained to up to8of its neighbors by spring forces.This application is representative of n-body dynamics simulations•Fractal-A shader that computes a40-iteration approx-imation to the Mandelbrot set.The shader is vectorized to process4adjacent points at once.This application waschosen to demonstrate that even shaders with a single out-put value may have strongly coupled intermediate values, and thus can benefit from hardware support for multiple outputs.•Matrix-A shader that computes an8by8dense matrix multiply.Input and output matrices are packed with two float4values per row.This shader shows how the merg-ing algorithms,while not parameterized by the number of outputs supported in hardware,can partition shaders that use more than the number of supported outputs.•Fire-A procedural volumetricfire shader[Ura02].This shader uses5octaves of4-dimensional Perlin noise,and demonstrates an extremely large shading computation with many levels of texture indirection.Table1shows the results of partitioning and executing our applications under each of our partitioning strategies.These tables show the number of passes in each partition,along with its cost as given by our metric.The execution timing results represent the average per-fragment execution time, in nanoseconds,when running the partitioned shader on the graphics hardware.These values were generated by amortiz-ing the time taken to shade a1024by1024pixel quad in the case of Particle and Fractal,and a512by512pixel quad in the case Matrix and Fire.All timing results were averaged over1000iterations.The compilation times represent the to-tal time spent in the partitioning algorithm,including time spent waiting on the external compiler.5.DiscussionPartitions generated by MRDS and MRDS outperform those generated by RDS for all of our shaders,including those that write only a single output.The relative increase in performance varies across the applications,and we will discuss these variations individually.Although the Particle shader writes multiple output val-ues,compiling for hardware with MRT support does not sig-nificantly increase its performance over a partition generated for single-output hardware.The majority of the instructions in the shader are used to calculate a sum of neighbor forces, and this running sum can be maintained with a single out-put per pass.Thefinal integration of position and velocity is the only step that generates multiple values.Despite a33% decrease in the number of passes,the merged partitions take only5%less time to execute.This result indicates that per-pass overhead is not the dominant factor in shader perfor-mance.The Fractal shader,despite writing only a single output value,shows a marked improvement in execution time when partitioned with MRDS or MRDS rather than RDS.The two merged partitions execute47%more quickly than the RDS partition.We attribute this result to the fact that the Frac-tal shader maintains two live values throughout most of its body.These values are used in an iterative computation,withc The Eurographics Association2004.。
- 1、下载文档前请自行甄别文档内容的完整性,平台不提供额外的编辑、内容补充、找答案等附加服务。
- 2、"仅部分预览"的文档,不可在线预览部分如存在完整性等问题,可反馈申请退款(可完整预览的文档不适用该条件!)。
- 3、如文档侵犯您的权益,请联系客服反馈,我们会尽快为您处理(人工客服工作时间:9:00-18:30)。
a r X i v :1102.5135v 1 [c o n d -m a t .m e s -h a l l ] 25 F eb 2011Localized States and Resultant Band Bending in Graphene Antidot SuperlatticesMilan Begliarbekov 1,Onejae Sul 2,John J.Santanello 1,Nan Ai 1,Xi Zhang 1,Eui-Hyeok Yang 2,and Stefan Strauf 11Department of Physics and Engineering Physics,Stevens Institute of Technology,Hoboken NJ,USA and2Department of Mechanical Engineering,Stevens Institute of Technology,Hoboken NJ,USA We fabricated dye sensitized graphene antidot superlattices with the purpose of elucidating the role of the localized edge state density.The fluorescence from deposited dye molecules was found to strongly quench as a function of increasing antidot filling fraction,whereas it was enhanced in unpatterned but electrically back-gated samples.This contrasting behavior is strongly indicative of a built-in lateral electric field that accounts for fluorescence quenching as well as p-type doping.These findings are of great interest for light-harvesting applications that require field separation of electron-hole pairs.Graphene,a two dimensional monolayer of carbon atoms arranged in a hexagonal lattice has been recently isolated [1]and shown to exhibit excellent electrical [2,3],thermal [4],mechanical [5]and optical [6]properties.Electron transport has been studied extensively in single and few-layer graphene sheets [7,8],while optoelectronic properties and light matter interaction in nanostructured graphene gain increasingly more interest in the research community,in particular since the advent of first ultra-fast graphene photodetectors [9].Single layer graphene absorbs only 2.3%of the incident radiation in the visible spectrum [10],consequently,efficient photocarrier sepa-ration within graphene becomes particularly important.In order to create a built-in electrical field that facil-itates carrier separation silicon based technology relies on the pn-junction that is created by doping the silicon lattice.Physical doping of graphene has been previously achieved by addition of extrinsic atomic [11,12]or molec-ular [13,14]species either by adsorption or intercalation into the graphene lattice [12,15].A potentially simpler way to make graphene a viable material for optoelec-tronics can be achieved by utilizing lateral electric fields created by Schottky barriers near the source and drain metal contacts [9,16,17],as was previously done in car-bon nanotubes [18].In the presence of such metal con-tacts it was also observed that nanotube fluorescence can be significantly enhanced [19].While graphene does not display any exciton emission,quantum dots placed on unpatterned graphene were recently shown to undergo strong fluorescence quenching,which is indicative of en-ergy transfer from the quantum dot exciton oscillator into graphene [20].Such hybrids between graphene and light harvesting molecules can potentially overcome the low absorption efficiency of bare graphene.Nanostructured graphene offers further possibilities to explore light harvesting and carrier separation.Of par-ticular interest are the so called antidot superlattices,i.e.,lattices comprized of a periodic arrangement of perfora-tions in the underlying graphene structure.These super-lattices were predicted to posses a nonnegligible magnetic moment [21],a small band gap [22–25]that can be con-trolled by the antidot filling fraction [26,27],and Peierls type electron-hole coupling that leads to polaronic be-Figure 1:(a)The energetic shift (black diamonds)and broad-ening (blue triangles)of graphene’s G-band as a function of the antidot filling fraction.(b)Positive correlation of the en-ergetic shifts of the G’and G bands on different mono,bi,and tri layer samples,showing effective p-doping.(c)Example SEM images of antidot lattices with different filling fraction.havior [26].In a previous work,Heydrich et al.,showed that the introduction of an antidot superlattice results in the stiffening of the G-Band in Graphene’s Raman spec-trum,as well as an energetic shift of the G and G’-Bands commensurate with p-type doping [28].Furthermore,re-cent theoretical predictions show that the periphery of graphene possesses a nonnegligible density of states N edge that is spatially localized at the edges and is distinct from the bulk states N bulk that are present in graphene’s inte-rior regions.Consequently,antidot superlattices provide a natural framework for studying these states and their properties,since the edge states in these systems coex-ist with the bulk states,unlike in dot lattices,where the ratio of edge to bulk states is small.Here we report an electro-optical study of dye sensi-tized graphene antidot superlattices with the purpose of elucidating the role of the localized edge state den-sity on its light-harvesting properties.The amount of2p-type doping introduced by the edge states is quanti-fied for various antidot filling fractions using confocal µ-Raman spectroscopy and transport measurements.We show that the fluorescence from deposited dye molecules strongly quenches in linear proportion to the antidot fill-ing fraction,whereas it was enhanced in the presence of free carriers in unpatterned but electrically back-gated samples.This contrasting behavior is strongly indica-tive of a built-in lateral electric field that accounts for fluorescence quenching as well as p-type doping and the observed Raman signatures.Our study provides new in-sights into the interplay of localized edge states in antidot lattices and the resulting band bending,which are critical properties to enable novel applications of nanostructured graphene for light harvesting and photovoltaic devices.I.RESULTS AND DISCUSSION A.Antidot SuperlatticesGraphene flakes used in these experiments were pre-pared by micromechanical exfoliation of natural graphite onto a degenerately doped p ++Si wafer with a thermally grown 90nm SiO yer metrology was subse-quently performed using confocal µ-Raman spectrometryin order to identify mono,bi,and tri-layer graphene flakes [29,30].Following the initial characterization,various antidot superlattices were etched onto the flakes using electron beam lithography.Figure 1c shows two exem-plary lattices with different filling fractions F =φ/s of antidots,where φis the antidot diameter,and s is their separation.In accordance with previous experimental re-sults [28,31,32],the corresponding Raman spectra dis-play an energetic shift and linewidth narrowing of the G-band with increasing filling fraction,as shown in Fig 1a.The G band,which occurs at ~1580cm −1arises from doubly degenerate iTO and iLO phonon modes which possess E 2g symmetry.The observed stiffening (from 16.7cm −1to 6.6cm −1)can be understood in terms of the Landau damping of the phonon mode,while the en-ergetic shift arises from a renormalization of the phonon energy [31,33].Furthermore,the energetic shift of the G-band is positively correlated with the shift of the G’-band,as shown in Fig.1b,which is indicative of an ef-fective p-doping of the underlying graphene layer[34,35].In contrast,a negative correlation in the energetic shifts of the G and G’bands would imply n-doping.In order to correlate shift and stiffening of the G-band in antidot superlattices to an underlying carrier density,we fabricated electrically contacted devices without an antidot lattice,as shown schematically in ing the electrical field effect of the back gate,the sheet car-rier density ∆n s was modulated and the stiffening and energetic shift of the G-band in the unpatterned samples was used to estimate the edge state density in the anti-dot superlattice (see supporting online material).From these data the amount of p-doping in the antidot samplesFigure 2:(a)Schematic of the spatially resolved confocal µ-Raman experiment,showing the excitation beam (λ0=532nm)and the three optical signals,R6G Raman,R6G fluo-rescence and graphene Raman,that were monitored during these experiments in both,electrically gated and antidot de-vices.(b)An example of an electrically contacted graphene device used in these experiments.was determined to reach up to 4×1012cm −2at a filling fraction of two (top axis in Fig.1a),and was not found to depend on the number of graphene layers as shown in Fig.1b.The large amount of effective p-doping is rather remarkable since neither extrinsic dopants,nor an exter-nal gate potential were applied to the antidot samples.Furthermore,in order to investigate the microscopic origin of the observed p-doping we fabricated graphene-dye hybrids.Both,antidot flakes and electrically con-tacted devices were soaked in a 15nanomol solution of Rhodamine 6G (R6G),as shown schematically in Fig.2a.In these experiments,the R6G Raman peaks,the R6G fluorescence,and the Raman signal from graphene were monitored as a function of the antidot filling fraction F as well as different backgate and source-drain biases on the unpatterned flakes.In the subsequent discussion,we first focus on the R6G fluorescence signal.Figure 3a shows a scanning electron micrograph of a single bilayer graphene flake with three distinct antidot superlattices L1,L2,and L3,which was used to study the spatially resolved µ-fluorescence of the R6G dye.The rel-ative intensity of the broad fluorescence signal of the R6G molecule (recorded at λF L =577nm)normalized to the intensity of R6G fluorescence on the bare SiO 2substrate are identified by circles in Fig.3a.Our results indicate that the R6G fluorescence is moderately quenched on the unpatterned graphene substrate as compared to the flu-orescence on the bare SiO 2wafer.Remarkably,the flu-orescence becomes even stronger quenched in the region were the antidot superlattices are located.The amount of R6G fluorescence quenching increases with increasing filling fraction of the antidots as shown in Figures 3b-3e for filling fractions of zero (graphene),1/3(L3),1/2(L2),and 1(L1).The integrated intensity of the R6G fluorescence signal quenches up to a factor of five for the largest realized filling fraction,as shown in Fig 4.In contrast to the quenching fluorescence signal,the intensity of the Raman signals from both R6G and graphene were found to increase six-fold with increas-ing filling fraction,i.e.increasing density of edge states,Figure 3:(a)Scanning electron micrograph of the graphene flake,with nanopatterned areas outlined by the green boxes.The filling fractions for lattice L1,L2,and L3are 1,1/2,and 1/3respectively (all dots are 100nm in diameter),the colors correspond to the R6G fluorescence in the sampling region normalized to the fluorescence on the bare SiO 2wafer.SEM’s of the individual lattices are available in the supporting online materials.Several example spectra taken on (b)lattice 1,(c)lattice 2,(d)lattice 3,and (e)unpatterned graphene,are alsoshown.Figure 4:Integrated intensity of the fluorescence signal (pink triangles)right axis,and R6G Raman signals taken at 1390cm −1(black squares)and 1630cm −1(green stars)left axis,as a function of the antidot filling fraction;as shown in Fig. 4.In order to rule out any possi-ble influence of the carboxylic bonds at the edges of the antidots and the possible presence of oxygen groups on SiO 2,which could have been introduced during oxygen plasma etching,a control experiment was performed in which several antidot lattices were reduced using 1mmolL-ascorbic acid for 24hours.Reduction in ascorbic acid was previously shown to effectively remove oxygen groups from graphene [36,37].Our results (which are shown in the supporting online materials)indicate that no signifi-cant oxygen contamination occurs during the 10s etching process,and thus cannot be used to account for the ob-served enhancement of the Raman peaks.Phenomenologically,the fluorescence quenching may be understood as follows.The incident laser light creates electron-hole pairs in the R6G dye.In the absence of the graphene substrate,the e-h pairs radiatively recombine thereby giving rise to the fluorescence signal on the bare SiO 2wafer.It was previously shown that placing quan-tum dots on top of graphene results in an energy transfer from the dots into the underlying graphene layer [38],re-sulting in a suppression of blinking from the quantum dots.A similar effect is expected to occur for the R6G molecules on graphene,where the radiative recombina-tion of the excitons in the R6G molecule is suppressed.In our experiments,additional quenching of the fluorescence signal in the antidot regions was observed (as shown in Figs.3and 4).The additional quenching can thus be understood to arise from the extra states at the edges N edge ,that effectively prevent radiative recombination of the electron-hole pairs,and therefore quench the flu-orescence signal.The amount of quenching observed in our experiments is rather remarkable since increasing the antidot filling fraction decreases graphene’s surface areaFigure5:(a)Gate tunable R6Gfluorescence of an unpatterned,electrically contacted device,similar to the one shown in Fig.2b,and(b)intensities of several Raman peaks(green stars taken at1630cm−1and black squares taken at1390cm−1), graphene’s G-band(red circles)and R6Gfluorescence(pink triangles).The blue curve shows the source-drain current I sd,that was used to determine the sheet carrier density∆n s(top axis)measured in a separate transport experiment in the same sample prior to the addition of R6G.and introduces larger areas of SiO2into the excitationvolume on which thefluorescence is not quenched.The observed linear increase in carrier density withincreasingfilling fraction is in accordance with the theo-retical prediction of Whimmer et al.[21],who showedthat the ratio of edge states to bulk states is givenby N edgeE20sR,where is the reducedPlanck’s constant,v F is the Fermi velocity in graphene,αis a parameter that characterizes edge roughness,E0is the energy width of the band of edge states,s is the an-tidot separation,and R is the antidot radius.Therefore, decreasing s or alternatively increasing R gives rise to alinear increase in N edge.B.Gate-Tunable FluorescenceIn order to further elucidate the mechanism forfluo-rescence quenching and the nature of N edge we fabricatedelectrically contacted and back-gated grapheneflakes, which did not contain an antidot superlattice.Varryingthe backgate voltage,effectively moves the Fermi level in the device thereby affording the possibility of in-situelectron and hole doping of the grapheneflake according to∆n s=C g(V g−V Dirac)/e,where C g is the gate ca-pacitance,V g is the applied gate voltage,V Dirac is the location of the Dirac point,and e is the electron charge[3,39–41].Modulating the Fermi level with the back-gate creates a free sheet carrier density in the underlying graphene layer.The effect of free carriers on the R6G fluorescence and the R6G and graphene Raman is shown in Fig.5a,with the blue(red)traces corresponding to spectra from hole(electron)doped regions and the black trace was taken at the Dirac point.The intensities of several Graphene and R6G Raman peaks are plotted in Fig.5b together with the I sd−V bg trace(blue line), which illustrates that the current to the left of the mini-mum(the Dirac point)is due to hole conductivity,while the current to the right of the minimum corresponds to electron conductivity.As can be seen,the intensities of both the Raman peaks as well as thefluorescence signal can be either quenched or enhanced by the applied gate bias,and directly follow the free carrier density in the paring the values of∆n s(top axis in Fig. 5b)to N edge(top axis in Fig.4)it is evident that the enhancement of the Raman peaks achieved in antidot de-vices occurs at comparable concentrations of N edge and sheet carrier densities∆n s in unpatterned samples,as shown in Figs.6a and6b.Unlike the Raman peaks,the R6Gfluorescence is strongly quenched in the nanopat-terned samples,whereas it is enhanced in the electrically gated samples.The contrasting behavior of thefluores-cence signal is strongly indicative of the different nature of the carriers in the antidot superlattice as compared to unpatterned graphene,and can be used to establish a microscopic mechanism for the observedfluorescence quenching and p-doping in the nanostructured samples.In principle,two possible mechanisms could be respon-sible forfluorescence quenching:charge transfer from R6G into the trap states that are created by the ad-ditional edge state density or electricalfield dissociation of the radiative R6G exciton,which leads to a strong decrease in the exciton recombination rate due to the re-duced electron-hole wavefunction overlap in an electric field.Although charge transfer into trap states could account for the decrease of thefluorescence intensity,it cannot explain the observed stiffening and the energetic shift of the G-band phonon in graphene,both of whichFigure6:(a)Comparison of thefluorescence quenching in the nanopatterned samples as a function of edge state carrier den-sity to(b)the enhancement offluorescence in gated samples in which free carriers are injected into the conduction band;(c)A schematic of the band bending that occurs as a results of pinning the Fermi level at the localized density of states ρedge at the edges of the antidots(orange dashed lines). require an electricfield effect[31,32].In contrast,the field dissociation mechanism explains both phenomena, as well as the absence offluorescence quenching in un-patterned graphene under back-gate sweeping.Since the edge states create spatially localized carriers, which are immobile,they would not cause the G-band stiffening.However,their presence effectively pins the Fermi level at the edges,thereby bending the band struc-ture throughout the entire antidot superlattice,since no localized states exist in graphene’s basal plane and the Fermi level must remain continuous,as shown schemati-cally in Fig.6c.This band bending creates an effective potential,i.e.a built-in lateral electricalfield,that ac-counts for the dissociation of the R6G excitons,resulting in the observedfluorescence quenching.In contrast,the vertical back-gatefield of the unpatterned graphene de-vice does not lead to band bending,while the created free carrier density can effectively feed the carrier cap-ture into the R6G molecules,causing the observedfluo-rescence enhancement.The Raman signals are enhanced by the electricalfield mechanism providing free carriers in both cases.Quantitatively,the effect of the built-in electricalfield may be estimated tofirst order from the amount of p-doping that it introduces.In graphene,doping is com-mensurate with the movement of the Fermi level into the conduction or valence bands by the electricalfield.The band offset∆E F as a function of doping concentration n is given by∆E F= v F k F,where is the reduced Planck’s constant,v F is the Fermi velocity,and k F is the Fermi wave vector,which in graphene is given by k F=√6Science320,5881(2008).[11]I.Gierz,C.Riedl,U.Starke,C.R.Ast,and K.Kern,Nano Letters8,4603(2007).[12]Y.Wang,Y.Shao,D.W.Matson,J.Li,and Y.Lin,ACSNano4,1790(2010).[13]T.O.Wehling,K.S.Novoselov,S.V.Morozov,E.E.Vdovin,M.I.Katsnelson,A.K.Geim,and A.I.Licht-enstein,Nano Letters8,173(2008).[14]X.Dong,D.Fu,W.Fang,Y.Shi,P.Chen,and L.-J.Li,Small5,1422(2009).[15]B.Guo,Q.Liu,E.Chen,H.Zhu,L.Fang,and J.R.Gong,Nano Letters In Press(2010).[16]T.Mueller,F.Xia,M.Freitag,J.Tsang,and P.Avouris,Phys.Rev.B79,245430(2009).[17]T.Mueller,F.Xia,and P.Avouris,Nature Photonics4,297(2010).[18]P.Avouris,Materials Today9,46(2006).[19]G.Hong,S.M.Tabakman,K.Welsher,H.Wang,X.Wang,and H.Dai,J.Am.Chem.Soc.132,15920 (2010).[20]Z.Chen,S.Berciaud,C.Nuckolls,T.F.Heinz,and L.E.Brus,ACS Nano4,2964(2010).[21]M.Wimmer,A.R.Akhmerov,and F.Guinea,Phys.Rev.B82,045409(2010).[22]J.Bai,X.Zhong,S.Jiang,Y.Huang,and X.Duan,Nature Nanotechnol.5,190(2010).[23]X.Liang,Y.-S.Jung,S.Wu,A.Ismach,D.L.Olynick,S.Cabrini,and J.Bokor,Nano Lett.10,2454(2010).[24]M.Kim,N.S.Safron, E.Han,M.S.Arnold,andP.Gopalan,Nano Lett.10,1125(2010).[25]A.Sinitskii and J.M.Tour,J.Am.Chem.Soc.132,14730(2010).[26]V.M.Stojanovic,N.Vukmirovic,and C.Bruder,Phys.Rev.B82,165410(2010).[27]R.Petersen and T.G.Pedersen,Phys.Rev.B80,113404(2009).[28]S.Heydrich,M.Hirmer,C.Preis,T.Korn,J.Eroms,D.Weiss,and C.Schuller,Appl.Phys.Lett.97,043113(2010).[29]M.Begliarbekov,O.Sul,S.Kalliakos,E.-H.Yang,andS.Strauf,Appl.Phys.Lett.97,031908(2010).[30]A.C.Ferrari,J.C.Meyer,V.Scardaci,C.Casiraghi,zzeri,F.Mauri,S.Piscanec,D.Jiang,K.S.N.S., Roth,et al.,Phys.Rev.Lett97,187401(2006). [31]J.Yan,Y.Zhang,P.Kim,and A.Pinczuk,Phys.Rev.Lett98,166802(2007).[32]S.Pisana,zzeri, C.Casiraghi,K.S.Novoselov,A.K.Geim,A.C.Ferrari,and F.Mauri,Nature Mate-rials6,198(2007).[33]A. C.Ferrari,Solid State Communications143,47(2007).[34]A.Das,S.Pisana,B.Chakraborty,S.Piscanec,S.K.Saha,U.V.Waghmare,K.S.Novoselov,H.R.Krishna-murthy,A.K.Geim,A.C.Ferrari,et al.,Nature Nan-otechnology3,210(2008).[35]C.Stampfer,F.Molitor,D.Graf,K.Ensslin,A.Jungen,C.Hierold,and L.Wirtz,Appl.Phys.Lett.91,241907(2007).[36]J.Zhang,H.Yang,G.Shen,P.Cheng,J.Zhang,andS.Guo,mun.46,1112(2010).[37]B.Krauss,P.Nemes-Incze,V.Skakalova,L.P.Biro,K.von Klitzing,and J.H.Smet,Nano Lett.10,4544 (2010).[38]Z.Chen,S.Berciaud,C.Nuckolls,T.F.Heinz,and L.E.Brus,ACS Nano4,2964(2010).[39]N.Stander,B.Huard,and D.Goldhaber-Gordon,Phys.Rev.Lett.102,026807(2009).[40]M.Begliarbekov,O.Sul,N.Ai, E.-H.Yang,andS.Strauf,Appl.Phys.Lett.97,122106(2010).[41]Y.-W.Tan,Y.Zhang,K.Bolotin,Y.Zhao,S.Adam,E.H.Hwang,S.D.Sarma,H.L.Stormer,and P.Kim,Phys.Rev.Lett.99,246803(2007).[42]E.Hwang,S.Adam,and S.D.Sarma,Phys.Rev.Lett.98,186806(2007).。