Peptide self-assembly in functional polymer science and engi
生物大分子自组装
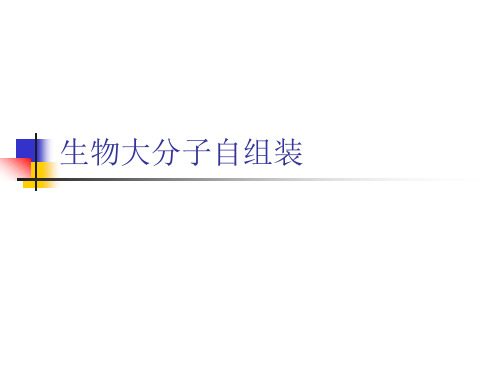
2.姜黄素
20个氨基酸 组成,赖氨 酸和缬氨酸 交替组成两 个臂,由于 赖氨酸带电 性质,静电 斥力作用多 肽折叠成一 个发夹,缬 氨酸具有疏 水性,发夹 与发夹之间 通过疏水性 与横向氢键 得到延伸。
3.海藻酸钠
由古洛糖醛酸(记为G酸)及其立体异构体甘露 糖醛酸(记为M酸)两种结构单元以三种方式 (MM段、GG段和MG段)通过α(1-4)糖苷键链接 而成的一种无支链的线性共聚物
2.生物大分子作为自组装材料有其天然的优越性,如 碱基互补配对、氨基酸识别等等,但目前为止,相关 研究并不充分,真正能应用的工业生产的材料几乎没 有
谢谢
生物大分子自组装
目录
1.引言 2. 原理 3.影响因素 4.表征手段 5.研究进展 6.应用 7.展望
引言
自组装(self-assembly):是指基本结 构单元(分子,纳米材料,微米或更大 尺度的物质)自发形成有序结构的一种 技术 。
在自组装的过程中,基本结构单元在基于非共价键的 相互作用下自发的组织或聚集为一个稳定、具有一定 规则几何外观的结构。
应用
主要用于纳米药物载体制备
主要包含蛋白质( 如明胶、白蛋白、丝蛋 白等) 和多糖( 如壳聚糖、海藻酸钠、环 糊精、果胶等) 两大类。
1.自组装肽/鞣质酸
双(N-乙酰氨基-苏氨酸) -1,5 - 戊烷二羧酸二甲酯
庚二酸(0.15克,0.94毫摩 尔),EDAC(0.05克,0.32 毫摩尔)和1 - 羟基苯并三唑 (0.05克,0.37毫摩尔)溶 解在DMF中,该混合物被冷却 至5℃并振摇1小时。然后加 入苏氨酸甲酯盐酸盐(0.3克 ,1.8毫摩尔),和三乙胺( 5升),5 ℃下搅拌24小时
生物信息学在蛋白质(酶)改造及设计中应用的新进展
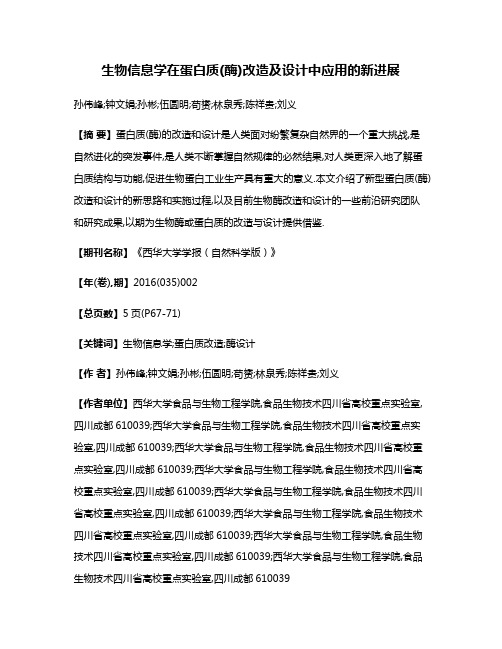
生物信息学在蛋白质(酶)改造及设计中应用的新进展孙伟峰;钟文娟;孙彬;伍圆明;苟赟;林泉秀;陈祥贵;刘义【摘要】蛋白质(酶)的改造和设计是人类面对纷繁复杂自然界的一个重大挑战,是自然进化的突发事件,是人类不断掌握自然规律的必然结果,对人类更深入地了解蛋白质结构与功能,促进生物蛋白工业生产具有重大的意义.本文介绍了新型蛋白质(酶)改造和设计的新思路和实施过程,以及目前生物酶改造和设计的一些前沿研究团队和研究成果,以期为生物酶或蛋白质的改造与设计提供借鉴.【期刊名称】《西华大学学报(自然科学版)》【年(卷),期】2016(035)002【总页数】5页(P67-71)【关键词】生物信息学;蛋白质改造;酶设计【作者】孙伟峰;钟文娟;孙彬;伍圆明;苟赟;林泉秀;陈祥贵;刘义【作者单位】西华大学食品与生物工程学院,食品生物技术四川省高校重点实验室,四川成都610039;西华大学食品与生物工程学院,食品生物技术四川省高校重点实验室,四川成都610039;西华大学食品与生物工程学院,食品生物技术四川省高校重点实验室,四川成都610039;西华大学食品与生物工程学院,食品生物技术四川省高校重点实验室,四川成都610039;西华大学食品与生物工程学院,食品生物技术四川省高校重点实验室,四川成都610039;西华大学食品与生物工程学院,食品生物技术四川省高校重点实验室,四川成都610039;西华大学食品与生物工程学院,食品生物技术四川省高校重点实验室,四川成都610039;西华大学食品与生物工程学院,食品生物技术四川省高校重点实验室,四川成都610039【正文语种】中文【中图分类】Q816从1965年首次获得溶菌酶(PDB:HEWL)晶体结构开始[1],人类就一直试图寻找和掌握蛋白质(酶)折叠成特定构象的奥秘。
从DNA水平、蛋白质的一级序列、二级结构到高级结构,人们一直在不断地探索以求掌握生命的秘密。
由于早期研究条件所限,仅能达到蛋白二级结构肽的设计水平,尽管如此,蛋白质(酶)改造和设计还是取得了丰硕的成果:研究者从7个氨基酸序列形成的α-helix出发,并在此基础上不断改进,成功设计了(helix-loop-helix- loop-helix)和α/β 折叠等新型蛋白[1],这些经典设计方法一直沿用至今;最近Koga等[2]在《Nature》杂志上报道了关于设计二级结构α-helix和β-sheet的一般规律。
用于软骨修复的水凝胶 - 浙江大学高分子科学与工程学系
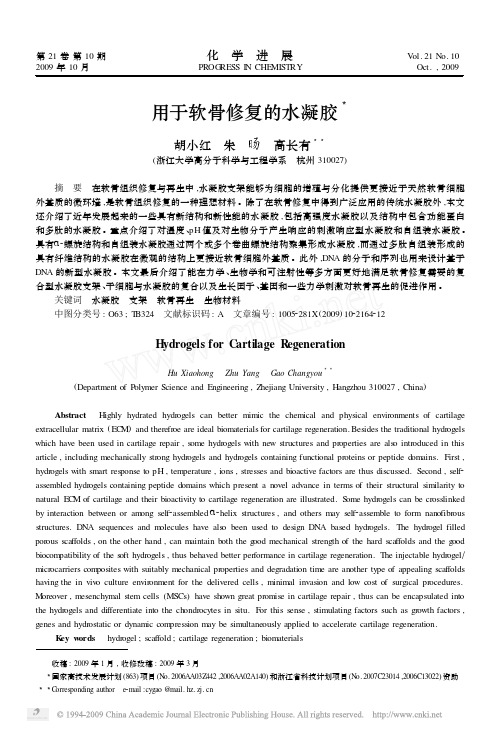
骨移植 。虽然这些方法成功地减轻了患者的痛苦 、 提高了软骨的功能 ,但是上述方法存在供体来源不 足 、手术过程复杂 、排异 、修复的软骨缺乏天然软骨 结构等缺点 。这些缺陷甚至可能阻碍这些治疗方法 在临床上的长期应用[3] 。
随着组织工程和再生医学技术的出现和逐步完 善 ,软骨修复技术出现了新的选择 。事实上 ,软骨修 复也是组织工程技术的最成功范例之一 。采用组织 工程技术修复软骨的过程一般是 :将体外分离扩增 的软骨细胞和生长因子或生物活性物质复合 ,然后 导入某种支架 ,再通过手术或微创注射的方法修复 缺损的软骨[4] 。除了种子细胞和活性因子外 ,支架 材料对于修复的软骨的质量起到至关重要的作用 。 除具有良好的机械物理性能外 ,更重要的是支架需 提供适于软骨组织再生的微环境[4] 。目前 ,已有包 括多孔支架 、纤维支架 、水凝胶和微载体在内的多种 结构的支架被用于软骨修复的研究和应用 。不同种 类的支架对软骨细胞的功能产生不同的影响 。由于 软骨细胞属于锚着依赖型细胞 ,它们在多孔支架和 微米纤维支架中需黏附在这些材料的表面才能生 长 ,通常呈现出铺展的扁平样形态[5] 。然而 ,软骨细 胞在纳米纤维支架和水凝胶支架中则成圆形或椭圆 形形态[5] ,这与其在天然软骨基质中更为接近 ,因而 更有利于维持软骨细胞的正常表型 。有研究表明 , 生长状态呈圆形或椭圆形的干细胞更倾向于向软骨 细胞分化[5] 。此外 ,水凝胶支架的水溶液环境更有 利于保护细胞以及易失活的药物如多肽 、蛋白质 、寡 聚核苷酸和 DNA 等 ,也有利于运输营养和细胞分泌 产物等 。由于水凝胶可以在一定条件下保持流动状 态而在外部物理或化学刺激下形成具有一定形状和 强度的体型材料 ,因此可以利用这种智能性来制备 注射型支架 ,发而 ,水凝胶也有机械强度 低 、消毒比较困难等缺点[6] 。近年来在水凝胶及其 复合物修复软骨方面已经取得了较大进展 ,并显示 出了良好的应用前景 。
肿瘤微环境-探针
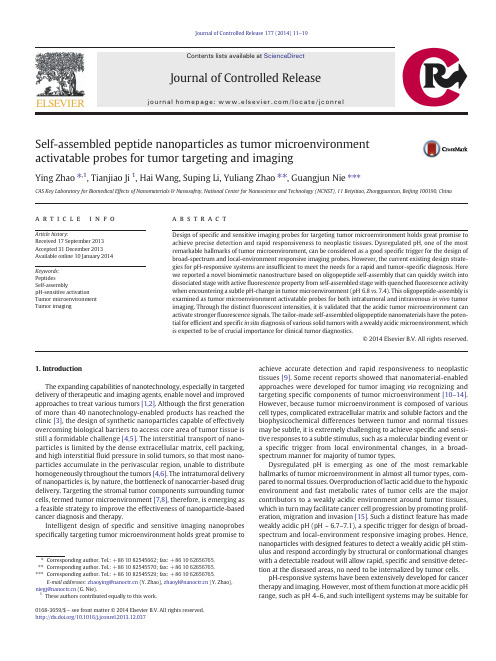
Self-assembled peptide nanoparticles as tumor microenvironment activatable probes for tumor targeting and imagingYing Zhao ⁎,1,Tianjiao Ji 1,Hai Wang,Suping Li,Yuliang Zhao ⁎⁎,Guangjun Nie ⁎⁎⁎CAS Key Laboratory for Biomedical Effects of Nanomaterials &Nanosafety,National Center for Nanoscience and Technology (NCNST),11Beiyitiao,Zhongguancun,Beijing 100190,Chinaa b s t r a c ta r t i c l e i n f o Article history:Received 17September 2013Accepted 31December 2013Available online 10January 2014Keywords:PeptidesSelf-assemblypH-sensitive activation Tumor microenvironment Tumor imagingDesign of speci fic and sensitive imaging probes for targeting tumor microenvironment holds great promise to achieve precise detection and rapid responsiveness to neoplastic tissues.Dysregulated pH,one of the most remarkable hallmarks of tumor microenvironment,can be considered as a good speci fic trigger for the design of broad-spectrum and local-environment responsive imaging probes.However,the current existing design strate-gies for pH-responsive systems are insuf ficient to meet the needs for a rapid and tumor-speci fic diagnosis.Here we reported a novel biomimetic nanostructure based on oligopeptide self-assembly that can quickly switch into dissociated stage with active fluorescence property from self-assembled stage with quenched fluorescence activity when encountering a subtle pH-change in tumor microenvironment (pH 6.8vs.7.4).This oligopeptide-assembly is examined as tumor microenvironment activatable probes for both intratumoral and intravenous in vivo tumor imaging.Through the distinct fluorescent intensities,it is validated that the acidic tumor microenvironment can activate stronger fluorescence signals.The tailor-made self-assembled oligopeptide nanomaterials have the poten-tial for ef ficient and speci fic in situ diagnosis of various solid tumors with a weakly acidic microenvironment,which is expected to be of crucial importance for clinical tumor diagnostics.©2014Elsevier B.V.All rights reserved.1.IntroductionThe expanding capabilities of nanotechnology,especially in targeted delivery of therapeutic and imaging agents,enable novel and improved approaches to treat various tumors [1,2].Although the first generation of more than 40nanotechnology-enabled products has reached the clinic [3],the design of synthetic nanoparticles capable of effectively overcoming biological barriers to access core area of tumor tissue is still a formidable challenge [4,5].The interstitial transport of nano-particles is limited by the dense extracellular matrix,cell packing,and high interstitial fluid pressure in solid tumors,so that most nano-particles accumulate in the perivascular region,unable to distribute homogeneously throughout the tumors [4,6].The intratumoral delivery of nanoparticles is,by nature,the bottleneck of nanocarrier-based drug delivery.Targeting the stromal tumor components surrounding tumor cells,termed tumor microenvironment [7,8],therefore,is emerging as a feasible strategy to improve the effectiveness of nanoparticle-based cancer diagnosis and therapy.Intelligent design of speci fic and sensitive imaging nanoprobes speci fically targeting tumor microenvironment holds great promise toachieve accurate detection and rapid responsiveness to neoplastic tissues [9].Some recent reports showed that nanomaterial-enabled approaches were developed for tumor imaging via recognizing and targeting speci fic components of tumor microenvironment [10–14].However,because tumor microenvironment is composed of various cell types,complicated extracellular matrix and soluble factors and the biophysicochemical differences between tumor and normal tissues may be subtle,it is extremely challenging to achieve speci fic and sensi-tive responses to a subtle stimulus,such as a molecular binding event or a speci fic trigger from local environmental changes,in a broad-spectrum manner for majority of tumor types.Dysregulated pH is emerging as one of the most remarkable hallmarks of tumor microenvironment in almost all tumor types,com-pared to normal tissues.Overproduction of lactic acid due to the hypoxic environment and fast metabolic rates of tumor cells are the major contributors to a weakly acidic environment around tumor tissues,which in turn may facilitate cancer cell progression by promoting prolif-eration,migration and invasion [15].Such a distinct feature has made weakly acidic pH (pH ~6.7–7.1),a speci fic trigger for design of broad-spectrum and local-environment responsive imaging probes.Hence,nanoparticles with designed features to detect a weakly acidic pH stim-ulus and respond accordingly by structural or conformational changes with a detectable readout will allow rapid,speci fic and sensitive detec-tion at the diseased areas,no need to be internalized by tumor cells.pH-responsive systems have been extensively developed for cancer therapy and imaging.However,most of them function at more acidic pH range,such as pH 4–6,and such intelligent systems may be suitable forJournal of Controlled Release 177(2014)11–19⁎Corresponding author.Tel.:+861082545662;fax:+861062656765.⁎⁎Corresponding author.Tel.:+861082545570;fax:+861062656765.⁎⁎⁎Corresponding author.Tel.:+861082545529;fax:+861062656765.E-mail addresses:zhaoying@ (Y.Zhao),zhaoyl@ (Y.Zhao),niegj@ (G.Nie).1These authors contributed equally to thiswork.0168-3659/$–see front matter ©2014Elsevier B.V.All rights reserved./10.1016/j.jconrel.2013.12.037Contents lists available at ScienceDirectJournal of Controlled Releasej o u r n a l h om e p a g e :w w w.e l s e v i e r.c o m /l o c a t e /j c o n r e lintracellular delivery(the acidic vesicles-mediated drug release),but not for tumor microenvironment triggered response(a subtle pH alter-ation)[16–20].Furthermore,most existing pH-sensitive nanoparticles based on long chain polymers showed a relatively slow response to pH stimulus from10h to several days[20–22].Therefore,these design strategies may be insufficient to meet the needs for a rapid and tumor environment-responsive diagnosis.As intelligent pH-responsive nano-particles,a rapid response is one of the most important advantages. Long response time may lead to their low tumor targeting efficacy due to re-entry blood circulation.Recently,some self-organized pH sensitive nanoparticles composed of polymers conjugated with pH-cleavable linkers or functional groups with pH-inducible charge conversion were designed[23–29],which showed certain sensitivity to the sub-tle changes in pH mimicking the difference between tumor microen-vironment and normal tissues.Despite some progress achieved,further development is urgently needed to consolidate the pH activatable strat-egy for tumor microenvironment targeting and imaging.For example, conjugating functional molecules atfixed location with controllable ratios and manner in polymer chains,and purification of the functional-ized polymers with biocompatibility and low or no toxicity are difficult to be executed.Self-assembled oligopeptide nanoparticles can address these chal-lenges.Firstly,oligopeptides are easily obtained by a solid-phase pep-tide synthesis(SPPS)method.Secondly,oligopeptides provide various reactive groups in the side chains for effective cross-linking and chemi-cal modification.Such rich chemistry for multi-functionality can be used for biomimetic or de novo designs to ensure the modification ratios and location and the product purity under tight stly,pH-sensitive disassembly of the oligopeptide nanostructure could render a rapid re-sponse to local stimuli compared to the long chain polymer nanoparti-cles,and the resulting low molecular weight constitutes can more readily diffuse within tumor tissues.Although extensive research efforts have been devoted to the design and fabrication of novel biomimetic nanomaterials through peptide self-assembly[30–33],tailored-design of intelligent oligopeptide nanostructures specifically recognizing and responding weakly acidic microenvironment(pH~6.7–7.1)of tumor tissues are challenging[34].We hypothesize that self-assembled nanostructures based on short peptides combined with chemical modifications with non-peptide functional groups may endow great opportunities for the design of tumor microenvironment rapidly responsive imaging probes with enhanced biocompatibility. The use of short peptides as the major building blocks to construct pH-activatable probes targeting tumor microenvironment through self-assembly process has,to the best of our knowledge,seldom been reported.Here we reported a novel biomimetic nanostructure based on oligopeptide self-assembly that can quickly switch into dissociated stage with activefluorescent property from self-assembled stage with quenchedfluorescence activity when changing local pH.An assembly/ disassembly was achieved by packing an energy pair(donor and recep-tor)into a peptide assembled nanostructure,a swift response from a self-assembled supramolecular nanoparticle(quenched state of the probes due to proximity of afluorescent dye and its quencher)into disassembled peptide molecules(activated state when encountering a pH trigger),which corresponds to a change in their surface charge (Fig.1).2.Materials and methods2.1.Materials3-Diethylaminopropyl isothiocyanate(DEAP),anhydrous di-methylformamide(DMF),N-methyl morpholine(NMM),and4-dimethylaminopyridine(DMAP)were purchased from Sigma-Aldrich (St.Louis,MO,USA).Alexa Fluor488Carboxylic Acid,Succinimidyl Ester(A488-SE)and black hole quencher-1(BHQ-1Carboxylic Acid,Succinimidyl Ester)were obtained from Life Technologies(Carlsbad, CA,USA)and Biosearch Technologies(Novato,CA,USA),respectively. Protected peptide conjugate KS5-DEAP2([CH3CONH]-K(DEAP)SKSK (DEAP)-[CONH2])was synthesized and purified by ChinaPeptides (Shanghai,China).Water used in the experiment was double-distilled (DW)and all other chemicals were used as received without any purification.2.2.Synthesis of A488and BHQ-1conjugated peptide KS5-DEAP2The peptide conjugates were prepared by conjugating pH-stable fluorescence dye,Alexa Fluor488(A488,ex/em:494nm/519nm) and BHQ-1(abs.534nm)to pH-sensitive peptide conjugate KS5-DEAP2.Peptide conjugate KS5-DEAP2([CH3CONH]-K(DEAP)SKSK (DEAP)-[CONH2])was synthesized using standard solid-phase Fmoc peptide chemistry.Then,A488-SE(12μmol)was coupled to the prima-ry amine of the central lysine residue on KS5-DEAP2(5μmol)in DMF (250μL)containing NMM(65μmol)and DMAP(3.2μmol)at room temperature in the dark under stirring for2h.The product(A-pep) was purified to N90%by reversed-phase high performance liquid chro-matography(RP-HPLC;Kromasil100-5C18,Eka Chemicals,Separation Products,SE-44580Bohus,Sweden):10%to50%acetonitrile containing 0.1%TFA versus DW containing0.1%TFA over20min at aflow rate of 4.0mL min−1.For quencher conjugation,the preparation process was similar with the above dye conjugation.Briefly,the BHQ-1(12μmol) was coupled to the primary amine of the central lysine residue on KS5-DEAP2(5μmol)in DMF(250μL)containing NMM(65μmol)and DMAP(3.2μmol)under stirring at room temperature in the dark over-night.The product(B-pep)was also purified by RP-HPLC.The mass of product was analyzed with Microflex LRF matrix-assisted laser desorp-tion ionization with time offlight(MALDI-TOF)mass spectrometer (Bruker Daltonics Inc.,USA).2.3.Preparation of hybrid peptide nanoparticles in aqueous solutionA-pep(5nmol)and B-pep(0,2.5,5,10nmol)were dissolved to-gether in DMF(1mL),and the solution was kept at room temperature with strong stirring for about1h.Subsequently,400μL DW was slowly added to the solution under strong stirring through a syringe within2h. The solution became slightly turbid,indicating the happening of self-assembly.After being stirred over night at room temperature,the solution was dialyzed against Tris·HCl buffer(pH7.4)under the room temperature to remove DMF for24h.The Tris buffer was changed every hour for thefirst6h,and subsequently every6h.The obtained solution wasfiltered and then set to10mL to get an A-pep concentra-tion about0.5μM.Finally,a series of products of hybrid peptide nano-particles(A/B ratio=2:1,1:1,1:2,and A-pep alone)was obtained.2.4.Dynamic light scattering(DLS)testThe particle size distribution of the products(A/B ratio=1:1)at pH7.4or pH6.8in Tris·HCl buffer was measured at room temperature by a ZetaSizer Nano series Nano-ZS(Malvern Instruments Ltd.,Malvern, UK)equipped with a He–Ne Laser beam at a wavelength of633nm and afixed scattering angle of90°.Prior to the test,the sample solution was stabilized at room temperature for2h.2.5.Transmission electron microscopy(TEM)observationThe morphology of the hybrid products was confirmed using a Transmission Electron Microscopy(Tecnai G2F20U-TWIN,FEI,USA). In brief,the samples were prepared by casting a solution of the hybrid peptide product(A/B ratio=1:1,pH7.4or6.8)on a copper grid, allowed to stand on the grid for90s.Filter paper was then used to wick away residual sample and liquid.One drop of1%(w/w)aqueous uranyl acetate(negative stain)was then placed on the grid,allowed to12Y.Zhao et al./Journal of Controlled Release177(2014)11–19stand for 20s,and subsequently removed by washing the grid with drops of DW and wicking away excess liquid with filter paper.The sam-ples were then dried in a vacuum oven for 24h.The morphology of the resulting samples was imaged using TEM.Prior to the test,the sample solution was stabilized at room temperature for 2h.2.6.Acid –base titration pro file measurementIn order to deeply understand the disassembly of the hybrid peptide nanoparticles resulting from DEAP dissociation,the p K b value of the peptide conjugated with DEAP was obtained by an acid –base titration method.The peptide conjugate (A/B ratio =1:1)dissolved in deion-ized water was adjusted to pH 10with 1M NaOH.The solution was titrated by the stepwise addition of 0.1M HCl solution to obtain the pH pro file.The average value from triplicate titrations was plotted.2.7.Zeta-potential measurementZeta-potential change of the products (A/B ratio =1:1)in Tris·HCl buffer at different pH values (pH 7.4,6.8,6.4,6.0)was measured with a ZetaSizer Nano series Nano-ZS (Malvern Instruments Ltd.,Malvern,UK).Prior to the test,the sample solution was stabilized at room temperature for 30min.2.8.Critical aggregation concentration (CAC)analysisThe stability of these nanoparticles was assessed by testing their CAC using pyrene fluorescence.The fluorescence properties of this molecule depend on the hydrophobicity of its environment,making it a sensitiveprobe for micelle aggregation.A 12mM solution of pyrene was pre-pared in ethanol,of which 1μL was added per 20mL of buffer to create a 6μM solution.The peptide conjugate (A/B ratio =1:1)was diluted serially into the pyrene-containing buffer.The intensity ratio of the first (370–373nm)to third (381–384nm)emission peaks in the pyrene fluorescence curve (334nm excitation)was monitored over a range of concentration at room temperature.This ratio was plotted over concentration,and the resulting curve showed a sigmoid.The CAC was taken as the in flection point of this curve,and this measure-ment was repeated for at least three dilution series.2.9.Fluorescence activation of hybrid peptide nanoprobesThe fluorescence activity change of the hybrid peptide probes was tested in 350μL quartz cuvette.The hybrid peptide nanoparticles (A/B ratio =2:1,1:1,1:2,and A-pep alone)in Tris·HCl buffer (pH 7.4)were stabilized at room temperature for 1h.After that,the pH was adjusted to 6.8,6.4,6.0,and 5.0with HCl.The amount of HCl was predetermined to ensure the final acidic pH values.Fluorescence signal intensity was detected with a LS55spectro fluorometer (Perkin Elmer Instruments,USA)at different time intervals.The excitation wavelength was fixed at 494nm.2.10.Fluorescence in vivo imagingFor the in vivo animal experiments,B16tumor cells were introduced into BALB/c nude mice via the subcutaneous injection of 1×106cells suspended in PBS (pH 7.4).To directly investigate the responsive effects of the activatable nanoprobes in the tumor (~0.50cm 3),thepeptideFig.1.Schematic illustration of the hybrid peptide nanoparticles as pH activatable probes.At physiological pH,the probes are at a quenched state.Upon reaching the weakly acidic tumor microenvironment protonation occurs and the probes are activated,thereby achieving tumor imaging.13Y.Zhao et al./Journal of Controlled Release 177(2014)11–19nanoparticles(A/B ratio=1:1,50μL)were intratumorally injected into B16tumor-bearing nude mice(1.5mg/kg body weight).Thefluo-rescence images were measured at different time post injection on a Maestro TM imaging system(Cambridge Research&Instrumentation, USA).To study the in vivo distribution and tumor accumulation of the hybrid peptide-based activatable probes,the nanoparticle solution and physiological saline(50μL)were intravenously introduced into B16 tumor(~0.31cm3)-bearing nude mice via tail vein injection.Fluores-cence images of the nude mice were monitored over time.All experi-ments with mice followed the guidelines for experimental animals and were approved by the local animal welfare committee.2.11.Statistical analysisThe error bars of Fig.3A showed the maximal and minimal values from three different samples for each experimental time.In addition, the error bars of other data were performed using an analysis of stan-dard deviation from three tofive different samples for each experimen-tal time(Error bars,±s.d.;n=3–5).3.Results and discussion3.1.Self-assembly and characterization of hybrid peptide nanoparticlesIt is challenging to integrate a short peptide with pH-sensitivity,a fluorescent dye,and its corresponding quencher into a platform under precise control of self-assemble/disassemble nature upon a designated pH change.Specifically,we designed an oligopeptide ([CH3CONH]-KSKSK-[CONH2],named KS5),in which three primary amino groups on the lysine residues could be used for conjugation. The N-and C-terminus of the peptide were acetylated and amidated to eliminate terminal charges,which may complicate the self-assembly process.These modifications also provide resistance against exopeptidases which recognize the free amino-and carboxy-termini for in vivo applications[35].Peptide KS5was conjugated with functional 3-diethylaminopropyl isothiocyanate(DEAP)which has been applied for polysaccharide-based photodynamic therapy[22,26].Peptide-DEAP conjugate was synthesized by a SPPS method using standard Fmoc strategy on CLEAR-Amid resin,and was characterized using MALDI-TOF-MS,1H NMR and FTIR spectroscopy(Supplementary Mate-rial,Fig.S1–S3).The resulting conjugate was expected to be sensitive to subtle changes in pH,because the p K b of DEAP ranges from7.0to7.3 [36],and the apparent p K b of peptide conjugated with DEAP was around 6.9(Supplementary Material,Fig.S4),which is close to the pH range in tumor microenvironment.The protonation of side chains at a weakly acidic pH should lead to a reduction in hydrophobicity,which triggers a pH-induced“intelligent switch”for the peptide self-assembled nano-structures,subsequently leading to their disassembly upon subtle changes of local pH.Fluorescent Alexa Fluor488(A488),a stable dye over a broad range of pHs,and black hole quencher-1(BHQ-1),a widely utilized quencher of A488,were coupled with peptide-DEAP conjugate to form two sets of hybrid peptide building blocks.It was therefore possible to obtain hybrid peptides with a ratio of one dye/quencher to one peptide,confirmed by mass and HPLC analysis(Supplementary Material,Fig.S5–S7).We then mixed the two types of peptide building blocks for self-assembly into nanoparticles,with vesicle structure in aqueous solution at neutral pH.The hydroxyl groups of serine residues were on the hydrophilic outer and inner surfaces,and the hydrophobic DEAP,A488and BHQ-1blocks were within the membrane bilayer (Supplementary Material,Fig.S8),in a hybrid fashion(Fig.1).Upon encountering the tumor microenvironment,the self-assembled nano-particles would undergo conformational changes and disassemble into the dispersive peptide building blocks.It is well known that the extracellular pH in most solid tumors is more acidic(pH~6.7–7.1)than in normal tissues(pH~7.4)[37]. Therefore,pH6.8was chosen in the following studies.The unique self-assembly/disassembly nature of the nanoparticles was validated experimentally(Fig.2).The magnitude of the size changes was dramatic between pH7.4(simulating normal tissue)and pH6.8(simulating tumor microenvironment).The self-assembled nanoparticles were formed with a diameter range from50to100nm at pH7.4,while less than5nm at pH6.8(Fig.2A and B),measured by DLS.Even a0.6pH unit dropped from7.4to6.8which resulted in disassembly of the nano-particles.Fig.2C showed that the nanostructure was almost spherical in shape at neutral pH,and the vesicle structure was also observed(insert in Fig.2C).However,it turned into a disassembled state at acidic pH,and very few aggregates were observed in this case(Fig.2D).The average diameters of nanoparticles measured by DLS were slightly larger than that of TEM,attributed to DLS measurement of hydrodynamic size of these nanoparticles[38].Moreover,the zeta potential of the nanostruc-tures revealed a change from negative to positive charge as the pH of the solution decreased from neutral to acidic.The negative charge at pH7.4 might originate from the hydroxyl groups,and was offset by the proton-ation of the DEAP block at pH6.8(Fig.2E).We also investigated the stability of the nanoparticles by measuring the critical aggregation concentration(CAC)using the pyrenefluorescence method[4].The CAC values of the peptide conjugates(A/B ratio=1:1)were0.15μM at pH7.4and57.23μM at pH6.8.The pretty small value at neutral pH indicated the potential application of the peptide nanoparticles in a di-luted medium,such as bodyfluids.As expected,acidic pH significantly increased CAC and the decreased stability is consistent with the reduced hydrophobicity of the DEAP blocks in the acidic media.Our current pep-tide assemblies demonstrated that the structural and conformational sensitivity between the pH of simulated normal tissue and the tumor microenvironment might present a new route for the construction of mi-croenvironment responsive imaging probes activated by a pH-trigger (summarized in Fig.1).3.2.Development of a pH-activatedfluorescence nanoplatformWe assume that the conglomeration of both A488dyes and BHQ-1 quenchers in the hydrophobic domain of nanoparticles would induce a quenched state of the overall nanostructure.When the probes are ex-posed to a weakly acidic environment,the self-assembled nanoparticles will disassemble,leading to the release of A488with the anticipation of restoring itsfluorescence activity(Fig.1).To assess the feasibility of such a design,thefluorescence changes of the hybrid peptide self-assembled nanoparticles were monitored byfluorospectrometry at dif-ferent pHs.Firstly,the two types of peptide-DEAP conjugates,coupled with A488(A-pep)and BHQ-1(B-pep),self-assembled into nanoparti-cles in aqueous solution at neutral pH and in the presence of serum (10%).Fig.3A showed that a dramatically lowfluorescent intensity was observed at pH7.4.This was attributed to the formation of self-assembled nanoparticles,which brought proximity of the quenching pairs and produced a quenched state from energy transfer among them.Subsequently,environmental pH was adjusted into acidic,and the amount of HCl was predetermined to ensure thefinal acidic pH6.8.We observed a dramatic increase influorescent activity (Fig.3A),which could be explained by a spatially disassociated state for the dyes and the quenchers of the peptide assemblies.Therefore, the capacity of the hybrid peptide self-assembled nanoparticles as activatable probes was confirmed.Additionally,our hybrid peptide nanoparticles showed a rapid pH-responsive rate.The activation curves in Fig.3A revealed a burst pH-responsive activation during30min.The composition of the hybrid nanoparticles,that is,the A-pep/B-pep (A/B)ratios in the nanostructures,may have an impact on the activa-tion capacity of probes.To optimize the formula,wefixed the A-pep concentration and varied the B-pep amounts to obtain a series of hybrid nanoparticles(A/B ratio=2:1,1:1,or1:2).A-pep alone was also investigated as a control.Fig.3A also showed that the hybrid nanoparticles with various A/B ratios were stable under the neutral con-ditions in the presence of serum(10%),and no obvious change of14Y.Zhao et al./Journal of Controlled Release177(2014)11–19fluorescent intensities was observed over the course of measurement (excitation at 494nm,emission at 520nm).This observation is expected as a design feature since all the A488dyes were covalently conjugated to the peptide-DEAP,and the structural and conformational stability of the nanoparticles at pH 7.4led to relatively stable fluorescent intensities.Obviously,despite all the hybrid nanostructures in a fluorescently quenched state,the composition signi ficantly in fluenced the quenching effect,with a higher BHQ-1composition showing a lower fluorescence intensity (intensities of 186,133,and 98a.u.for hybrid nanoparticles with a A/B ratio of 2:1,1:1,and 1:2,respectively,at t =0).A-pep alone also presented a certain degree of quenching effect (302a.u.at t =0),owing to the self-quenching effects among the A488fluorophores.A pH-dependent activation and composition-dependent fluores-cence quenching and activation were obtained for all the hybrid peptide probes including A-pep alone (Fig.3B).Particularly,the nanostructures with A/B ratios of 2:1and 1:1achieved an approximately full activation capacity after 60min of pH change,while only 54%recovery was shown for the one with the A/B ratio of 1:2.This might originate from the excess amount of BHQ-1molecules which blocked the disassembling process of nanoparticles (Supplementary Material,Fig.S9).The p K b values of peptide conjugates A-pep and B-pep were around 7.0and 6.5,respectively.Overall,the hybrid probe with an A/B ratio of 1:1showed the highest fold activation (about 6-fold under the described conditions in Fig.3B),so we selected it for further experiments.Toobtain a series of activation patterns at various acidic pH,the incubation solutions were then adjusted into even more acidic pHs (6.4,6.0,and 5.0),and fluorescent properties were also measured at these pHs.As shown in Fig.3C,the pH-dependent activation allowed higher fluores-cence restoration at lower pH.Especially,pH 6.0was acidic enough to activate the probes in 30min and further lowering pH achieved compa-rable activation capacity,as observed at pH 5.0.3.3.Dynamic process of nanoparticles disassemblyTo clarify whether the peptide nanoparticle disassembly followed the design strategy and to further con firm the time point when the quenched state of nanoparticles was completely transferred to the de-quenched fluorescent state,the morphology change of the peptide nanoparticles (A/B =1:1)was monitored by TEM at different time points after adjusting the environmental pH into 6.8(Fig.4).With increasing the time,the hierarchical disassembly and collapse of nano-particles were clearly observed.The disassembly was kinetically charac-terized by two phases:a transient swelling followed by a rapid collapse phase.The morphology of the nanoparticles changed from spherical to formless,and there were not apparent nanoparticles 60min after pH change.These TEM images strongly supported the conclusion that the nanoparticle disassembly was caused by the protonation of the DEAP block at pH 6.8,which resulted in the activation of the nanoparticles from a quenched state to the de-quenched fluorescent state.Atime-Fig.2.Characterization of the pH-sensitive hybrid peptide nanoparticles.Particle-size distribution of the formula (A/B ratio =1:1)at pH 7.4(A)and pH 6.8(B)measured by DLS.Transmission electron microscopy (TEM)images of the formula (A/B ratio =1:1)at pH 7.4(C)and pH 6.8(D).(E)Zeta-potential change was observed for the peptide conjugate products at different pH values (pH 7.4–6.0).15Y.Zhao et al./Journal of Controlled Release 177(2014)11–19。
自组装短肽水凝胶与骨组织构建

《中国组织工程研究》Chinese Journal of Tissue Engineering Research文章编号:2095-4344(2019)34-05531-075531·综述·www.CRTER .org熊娜,女,湖北省襄阳市人,汉族,遵义医科大学在读硕士,主要从事自组装短肽特性及组织工程方向的研究。
通讯作者:刘燕飞,博士,副研究员,硕士生导师,遵义医科大学附属医院贵州省细胞工程重点实验室,贵州省遵义市563003文献标识码:A稿件接受:2019-05-30Xiong Na,Master candidate,Key Laboratory of Cell Engineering in Guizhou Province,Affiliated Hospital of Zunyi Medical University,Zunyi 563003,Guizhou Province,China Corresponding author:Liu Yanfei,PhD,Associate researcher,Master’s supervisor,Key Laboratory of Cell Engineering in Guizhou Province,Affiliated Hospital of Zunyi Medical University,Zunyi 563003,Guizhou Province,China自组装短肽水凝胶与骨组织构建熊娜,刘燕飞,卫巍(遵义医科大学附属医院贵州省细胞工程重点实验室,贵州省遵义市563003)DOI:10.3969/j.issn.2095-4344.1446ORCID:0000-0001-6127-1953(熊娜)文章快速阅读:文题释义:自组装短肽水凝胶:由相互交替的亲水性和疏水性氨基酸序列组成,在一定条件(离子浓度、温度、pH 值等)刺激下能够自发且有规律地通过非共价键作用形成稳定的β折叠结构,再进一步堆叠为纳米纤维,纳米纤维形成交织基质,最终形成含水量超过99%的水凝胶支架。
self-assembly的生物学解释

自我组装(self-assembly)是指微观尺度上的分子或物质自发地按照特定的规律结合在一起形成有序的结构。
在生物学中,自我组装现象在细胞和生物体各个组织及器官中都有广泛的应用。
本文将从生物学的角度探讨自我组装的相关概念、机制和应用。
一、自我组装的概念自我组装是一种自发的过程,通过该过程,分子或物质在没有外部干预的情况下,按照一定的规律自行组合形成有序的结构。
这种自组装过程常常受到分子间的相互作用力的影响,比如静电相互作用、疏水相互作用等。
在生物体内,自我组装现象表现为细胞自组装形成组织、器官和生物体整体结构。
二、细胞内的自我组装1. 蛋白质自组装细胞内的许多结构和器官都是通过蛋白质自组装形成的。
细胞骨架是由微管和微丝等蛋白质组成的,它们按照特定的规律在细胞内自我组装形成细胞骨架,维持细胞的形态和结构。
2. 膜蛋白的自组装细胞膜是由多种膜脂和膜蛋白组成的,它们在细胞内通过自组装形成双分子层结构,保护和维持细胞的完整性。
三、生物体的自我组装1. 胚胎发育过程中的自我组装在生物体的胚胎发育过程中,不同的胚层细胞会按照特定的规律自我组装形成各种器官和组织,最终构成一个完整的生物体。
2. 组织和器官的自我组装在成体生物中,细胞和组织按照一定的规律自我组装,形成不同的器官和系统,保持生物体的结构和功能。
四、自我组装的应用1. 生物医学领域利用自我组装原理可以设计和制造新型的生物材料,用于组织工程和再生医学等领域,有望为医学治疗和健康管理带来新的突破。
2. 纳米技术领域自我组装原理被广泛应用于纳米技术领域,用于设计和制备纳米材料和纳米器件,有望推动纳米技术的发展和应用。
五、总结自我组装作为一种重要的生物现象,不仅在细胞和生物体内起着重要的作用,而且在生物医学和纳米技术等领域也具有重要的应用价值。
深入研究自我组装的机制和规律,将有望为生物学和生物技术领域带来新的发展机遇。
六、自我组装的机制1. 分子间相互作用在生物体内,自我组装的过程受到分子间相互作用的影响。
聚合物胶束的稳定性及影响因素
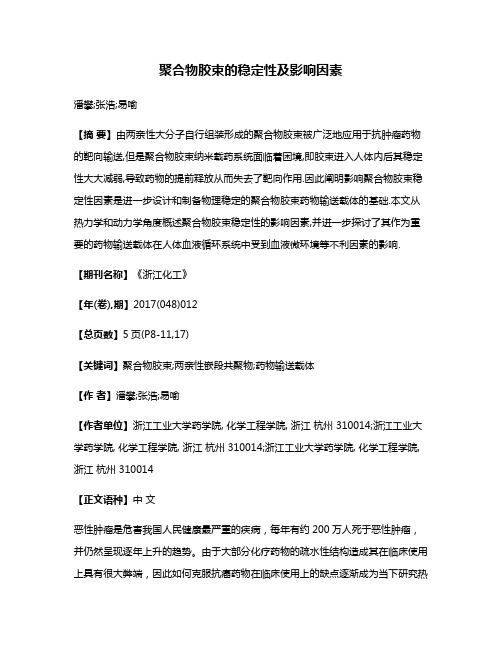
聚合物胶束的稳定性及影响因素潘攀;张浩;易喻【摘要】由两亲性大分子自行组装形成的聚合物胶束被广泛地应用于抗肿瘤药物的靶向输送,但是聚合物胶束纳米载药系统面临着困境,即胶束进入人体内后其稳定性大大减弱,导致药物的提前释放从而失去了靶向作用.因此阐明影响聚合物胶束稳定性因素是进一步设计和制备物理稳定的聚合物胶束药物输送载体的基础.本文从热力学和动力学角度概述聚合物胶束稳定性的影响因素,并进一步探讨了其作为重要的药物输送载体在人体血液循环系统中受到血液微环境等不利因素的影响.【期刊名称】《浙江化工》【年(卷),期】2017(048)012【总页数】5页(P8-11,17)【关键词】聚合物胶束;两亲性嵌段共聚物;药物输送载体【作者】潘攀;张浩;易喻【作者单位】浙江工业大学药学院, 化学工程学院, 浙江杭州 310014;浙江工业大学药学院, 化学工程学院, 浙江杭州 310014;浙江工业大学药学院, 化学工程学院, 浙江杭州 310014【正文语种】中文恶性肿瘤是危害我国人民健康最严重的疾病,每年有约200万人死于恶性肿瘤,并仍然呈现逐年上升的趋势。
由于大部分化疗药物的疏水性结构造成其在临床使用上具有很大弊端,因此如何克服抗癌药物在临床使用上的缺点逐渐成为当下研究热点[1]。
聚合物纳米胶束由于其亲水外壳-疏水内核的结构可以对疏水性的抗癌药物进行包封装载,一方面可以增大药物在体内的水溶性,另一方面减小药物对人体的毒副作用[2]。
目前聚合物胶束药物载体与其它纳米药物面临同样的困境,即在一定程度上可以减轻毒副作用,但是疗效较原药并没有显著的提高。
可能的原因是胶束在血液循环系统的快速解离导致了药物的提前释放从而失去了靶向性,造成胶束化药物体内疗效不高。
因此,阐明体内和体外环境下影响聚合物胶束稳定性的因素,将为进一步设计物理性质稳定的聚合物胶束进而获得高效胶束型纳米药物提供重要理论基础。
聚合物胶束是指两亲性嵌段共聚物(一端为亲水性聚合物,一端为疏水性聚合物)在溶液中达到一个临界浓度时,由分子间的氢键、范德华力和疏水相互作用等自发形成纳米级亲水性外壳,疏水性内核的核壳结构[3](如图1)。
生物材料英语单词合集
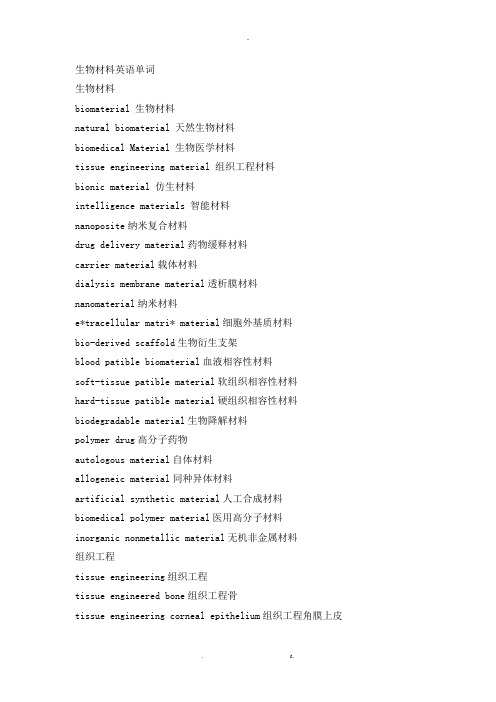
生物材料英语单词生物材料biomaterial 生物材料natural biomaterial 天然生物材料biomedical Material 生物医学材料tissue engineering material 组织工程材料bionic material 仿生材料intelligence materials 智能材料nanoposite纳米复合材料drug delivery material药物缓释材料carrier material载体材料dialysis membrane material透析膜材料nanomaterial纳米材料e*tracellular matri* material细胞外基质材料bio-derived scaffold生物衍生支架blood patible biomaterial血液相容性材料soft-tissue patible material软组织相容性材料hard-tissue patible material硬组织相容性材料biodegradable material生物降解材料polymer drug高分子药物autologous material自体材料allogeneic material同种异体材料artificial synthetic material人工合成材料biomedical polymer material医用高分子材料inorganic nonmetallic material无机非金属材料组织工程tissue engineering组织工程tissue engineered bone组织工程骨tissue engineering corneal epithelium组织工程角膜上皮vascular tissue engineering血管组织工程tissue engineering heart valve组织工程瓣膜tissue Engineered Medical Products组织工程医疗产品nerve tissue engineering神经组织工程tissue engineered cartilage组织工程软骨nanofiber scaffolds for liver tissue engineering肝脏组织工程纳米纤维支架vascularized tissue-engineered scaffold血管化组织工程骨支架tissue-engineered epidermis containing melanocyte含黑色素细胞的组织工程表皮tissue engineering models for cardiac muscle心肌组织的组织工程模型tissue-engineered tracheal epithelial cells组织工程化气管上皮细胞tissue-engineering skin scaffold material组织工程化皮肤支架材料fibers Tissue engineering scaffold纤维组织工程支架种子细胞seed cell种子细胞salivary gland seed cell颌下腺种子细胞interstitial seed cell间质种子细胞cartilage seed cell软骨种子细胞seed cell bank种子细胞库tendon seeding cell肌腱种子细胞embryonic stem cell胚胎干细胞nerve stem cell神经干细胞adult Stem Cell成体干细胞cancer stem cell肿瘤干细胞adipose-derived stem cell脂肪干细胞bone marrow mesenchymal stem cell骨髓间充质干细胞hepatic stem cell ; liver stem cell肝干细胞hematopoietic stem cell造血干细胞peripheral blood hematopoietic stem cell transplantation外周血造血干细胞移植pluripotential hematopoietic stem cell多能造血干细胞multipotential hematopoietic stem cell全能造血干细胞umbilical cord blood transplantation脐带血造血干细胞移植induced pluripotent stem cell诱导多能干细胞endothelial progenitor cell皮祖细胞材料表征方法rapidlyquenching快速凝固法severe(intense)plasticdeformation强烈塑性变形法amorphoussolidcrystallization非晶晶化法in-situposite原位复合法intercalationhybrids插层复合法microemulsion微乳液法templatesynthesis模板合成法self-assembly自组装法graphitearcdischarge石墨电弧放电法rapidlyquenching快速凝固法passivatingtreatment稳定化处理gas-condensatin method气体冷凝法liquid-phase method液相法solid-phase method固相法glucose-Fe ple* coating糖铁络合物涂层surface modification外表修饰改性layer-by-layer self-assembly层层自组装inert gas deposition惰性气体沉积法high energy ball mill高能球磨法freeze drying冷冻枯燥法hydrothermal synthesis水热合成法radiation chemical synthesis辐射化学合成法材料特性检测方法:field ion microscopy (FIM)场离子显微法magnetic force microscopy (MFM)磁力显微法laser interferometer激光干预仪laser diffraction and scattering激光衍射/散射法centrifugal sedimentation离心沉降法*-ray diffractometry (*RD)* 射线衍射法scanning probe microscopy (SPM)扫描探针显微镜infrared absorption spectroscopy红外吸收光谱法*-ray diffractometry line broadening (*RD-LB) * 射线衍射线宽化法small angle *-ray scattering (SA*S) * 射线小角度散射法raman spectrometry拉曼光谱法mossbauer spectrometry穆斯堡尔谱法photon correlation spectroscopy光子相关谱法mercury porosimetry压汞仪法nano impress纳米压痕仪scanning tunneling electron microscopy (STM)扫描隧道电子显微法scanning near-field optical microscopy (SNOM)扫描近场光学显微法atomic force microscopy (AFM) 原子力显微法scanning capacitance microscopy (SCM)扫描电容显微法scanning thermal microscopy (STHM)扫描热显微法材料特性fle*ural strength抗折强度tensile strength抗拉强度pressive strength抗压强度hyperelastic 超弹性finite element 有限元biopatibility生物相容性biomechanics生物力学缓释slow release;controlled-release缓释slow release inde*缓释指数sustained release pellet缓释丸sustained release sponge缓释明胶hydro*ycamptothecin Sustained-released Tablet羟基喜树碱缓释片sustained Release PLGA Microsphere PLGA生物可降解缓释微球slow-release pound Acidifier缓释复合酸化剂drug sustained-release hydrogel film水凝胶药物缓释膜脱细胞支架decellularized scaffold脱细胞支架acellular scaffolds matri*脱细胞支架材料homograft collagenous scaffold同种生物脱细胞支架decellularized scaffold of artery脱细胞动脉支架acellular vascular scaffold脱细胞血管支架cellularized nerve scaffold脱细胞神经支架acellular dermal scaffold脱细胞真皮支架decellularized vascular bioscaffold血管脱细胞生物支架acellular cartilage material脱细胞软骨支架材料detergent-e*tracted muscle scaffold脱细胞骨骼肌支架acellular spinal cord scaffold脱细胞脊髓支架移植transplantation移植organ transplantation器官移植transplanted organ移植器官kidney transplant肾脏移植heart transplant心脏移植transplant rejection移植排斥liver transplantation肝移植*enoplastic transplantation异种移植autoplastic transplantation自体移植bone transplantation骨移植corneal transplant角膜移植tissue transplantation组织移植fat transplantation脂肪移植graft-versus-host disease移植物抗宿主病transplantation antigen移植抗原纳米材料nanophase material纳米材料niobium-o*ide Nano-material铌氧化物纳米材料conductive Nano-material导电纳米材料one-dimensional nanomaterial一维纳米材料nanoposite复合纳米材料nano material Engineering 纳米材料工程nanometer material science; nanometer scale materials; Nanometer scale materials; nanometer materials science纳米材料科学functional nano material; functional nanomaterials功能纳米材料semiconductor nanomaterial; nano sized semiconductor material; nanometer-sized semiconductor materials; semiconductor nano-material 半导体纳米材料inorganic nano-material; inorganic nanometer material; nano-inorganic material; inorganic nano-material无机纳米材料nano materials Chemistry纳米材料化学进展doped nano materials掺杂纳米材料nanotechnology 纳米技术nanoparticle纳米颗粒nanocristal纳米晶体nano Zinc o*ide纳米氧化锌nanospheres纳米球nano-selenium纳米硒nanocrystalline纳米晶nanoscience;nanometer science纳米科学微球microsphere;microparticle微球micro-solder ball微锡球biological microcapsule生物微胶球micro-sphere target微靶球hollow glass micro-spheres空心玻璃微球球壳PLA microsphere聚乳酸微球Immunomagnetic Microsphere; Immuno-magnetic bead免疫磁性微球microspherolitic微球粒状的carbon microsphere碳微球β-Cyclode*trin Polymer Microsphere β-环糊精聚合物微球anion starch microsphere阴离子淀粉微球chitosan Microsphere壳聚糖微球octafluoropropane albumin microsphere八氟丙烷白蛋白微球polyelectrolyte posite Microsphere聚电解质复合微球gelatin microsphere明胶微球alginate microsphere海藻酸钙微球magnetic posite polymer microsphere复合型磁性高分子微球gelatin/ Hydro*yapatite posite Microsphere明胶/羟基磷灰石复合物微球porous Magnetic posite Microsphere多孔磁性复合微球magnetic polyvinyl acetate microsphere磁性聚醋酸乙烯酯微球支架scaffold支架bio-derived scaffold生物衍生支架decellularized scaffold去细胞化支架bio-derived scaffold生物衍生支架biomaterial scaffold 生物支架biological scaffold material生物支架材料 ;PLG scaffold PLG生物支架decellularized vascular bioscaffold血管脱细胞生物支架the creature valves frame生物瓣支架biodegradable stent生物降解支架biological carrier生物载体支架bioactive porous scaffold生物多孔支架胶原collagen胶原e*tracellular matri* 细胞外基质interstitial collagen 间质胶原basement membrane collagen 基膜胶原type I collagen I型胶原type Ⅱ collagen Ⅱ型胶原type Ⅲ collagen Ⅲ型胶原type Ⅴ collagen Ⅴ型胶原type Ⅺ collagen Ⅺ型胶原collagen fiber胶原纤维ceramide胶原因子soluble collagen可溶性胶原collagen Peptide胶原肽collagen casing胶原肠衣collagen synthesis胶原合成collagenases胶原酶类collagen sugar胶原糖bovine-based collagen牛胶原mature collagen fibrils 成熟胶原纤维collagen disease胶原性疾病collagen Biomineralization Reation胶原生物矿化反响collagen sheet胶原敷料collagen/ Chitosan posite Dispersion胶原/壳聚糖复合溶胀液biological collagen fiber生物胶原纤维e*ogenous collagen membrane异种胶原生物膜壳聚糖chitosan壳聚糖chitin 甲壳素sulfonated chitin 磺化甲壳素sulfonated carbo*ymethyl chitin 磺化羧甲基甲壳素hydro*yethyl chitosan 羟乙基壳聚糖acetylated Chitosan 乙酰化壳聚糖carbo*ymethyl chitosan 羧甲基壳聚糖iodine chitin 碘代甲壳素hydrolyzed chitosan 水解壳聚糖calciumphosphate∕chitosan coatings钙磷/壳聚糖涂层oligo-chitosan壳低聚糖chitooligosaccharide壳寡聚糖oligochitosan-Cu壳低聚糖铜配合物chitooligosaccharide-cysteine壳低聚糖-半胱氨酸衍生物chitosan film壳聚糖膜glycol chitosan乙二醇壳聚糖chitosan conduit壳聚糖导管chitosan/ tricalcium phosphate壳聚糖-磷酸三钙复合材料natural Rubber/ Carbo*ymethyl-Chitosan Antibacterial posite天然橡胶/羧甲基壳聚糖抗菌复合材料chitosan-alginate microcapsule壳聚糖-海藻酸钠微囊chitosan derivation壳聚糖衍生物quaternized Chitosan壳聚糖季铵盐pH-sensitive chitosan/ gelatin hydrogel pH敏感性壳聚糖/明胶水凝胶pva/ water-soluble chitosan/ glycerol hydrogel聚乙烯醇/水溶性壳聚糖/甘油水凝胶polycation-modified Chitosan Material多聚阳离子修饰壳聚糖材料chitosan nanoparticle壳聚糖纳米粒thermosensitive Chitosan Hydrogel壳聚糖温敏性水凝胶多糖polysaccharide多糖capsular polysaccharide荚膜多糖core polysaccharide核心多糖acid polysaccharide酸性多糖tremella polysaccharides银耳多糖fungus polysaccharides食用菌多糖polysaccharides 聚多糖Polysaccharide Nano-particle聚多糖纳米粒natural Polysaccharide天然聚多糖high molecular weight polysaccharide高分子量聚多糖water-soluble Polysaccharose水溶性接枝聚多糖proteoglycans from the e*tracellular matri*细胞外基质蛋白聚多糖polysaccharide nanocrystals modified material聚多糖纳米晶改性材料natural gather cationic biological polysaccharide天然的聚阳离子多糖polyanion mucopolysaccharide聚阴离子粘多糖polygalacturonase多聚半乳糖醛酸酶水凝胶hydrogel; aquogel; lyogel 水凝胶aquagel fiber水凝胶纤维thermo-sensitive hydrogel温敏水凝胶PVA/ Glutin/ Startch Hydrogel PVA/明胶/淀粉水凝胶physical Cross-linking Polyurethane Hydrogel 物理交联型聚氨酯水凝胶polyacrylamide gel 聚丙烯酰胺水凝胶hydrogel bolster水凝胶衬垫poly ( N-acryloylglycine) hydrogels 聚N-丙烯酰基甘氨酸水凝胶pH-sensitive hydrogel pH值敏感的水凝胶thermosensitive Chitosan Hydrogel壳聚糖温敏性水凝胶smart hydrogel; Intelligent hydrogel智能水凝胶pH and Temperature Sensitive Starch Hydrogel pH值/温度双重敏感淀粉水凝胶polymeric hydrogel聚合水凝胶nanogel纳米水凝胶biodegradable pH-and temperature-sensitive hydrogel温度和pH双重敏感性可生物降解水凝胶AAm/ Ac hydrogel丙烯酰胺/丙烯酸水凝胶supramolecular hydrogel超分子水凝胶silicone hydrogel 硅水凝胶聚乳酸polylactic acid 聚乳酸poly-L-lactic acid聚左乳酸poly(lactide-co-glycolide)聚(乳酸-乙醇酸)poly(lactic acid-co-aspartic acid)聚(乳酸-天冬氨酸)polylactic acid fiber聚乳酸纤维poly(L-lactide) nano-fiber scaffold聚乳酸纳米纤维支架材料polylactic resin聚乳酸树脂金属材料metallic material金属材料biomedical metallic material生物医用金属材料Zr-Based Biomedical Alloy Zr基生物医用金属材料high property alloy steel 高性能合金钢Al-Li alloy铝锂合金magnesium alloy镁合金superalloy高温合金biodegradable metallic material可生物降解性金属材料stainless steel 不锈钢nickel-cobalt alloy镍钴铬合金carbon/ metal nanoposite纳米金属/碳复合材料the knitted medical e*pandable metallic stent针织医用金属支架biomedical porous metal生物医用多孔金属材料nickel titanium shape memory alloy镍钛形状记忆合金生物瓷biological ceramic生物瓷piezoelectric bioceramics压电生物瓷hydro*yapatite Bioceramics羟基磷灰石生物瓷biodegradable ceramics降解性生物瓷bioactive ceramics活性生物瓷absorbable bioceramics可吸收生物瓷bioinert ceramics惰性生物瓷bioceramic coatings生物瓷涂层bioceramics生物瓷学aluminium o*ide bioceramic氧化铝生物瓷电纺丝electrospining电纺丝electrospinning setups电纺丝设备blow-electro spinning气-电纺丝electrospinning technique电纺丝技术electrospinning installation电纺丝装置ABC-spinning高速气电纺丝electrospinning classification电纺丝分类coa*ial electrospraying(electrospinning)同轴电雾化(电纺丝)spinning machine spindle纺丝电锭electrospun silk fibroin/ poly(ε-caprolactone) ultrafine fiber membrane 电纺丝素蛋白/聚己酯超细纤维膜electrospun fibers drug formulation电纺丝超细纤维药物剂型electrospun fiber电纺丝纤维electrospun ultrafine fiber电纺丝超细纤维continuous Spinning of Piezoelectric PZT Ceramic Fibers PZT压电瓷纤维连续纺丝electrospinning solution 电纺丝液海藻酸alginic acid 海藻酸ammonium alginate海藻酸铵calcium alginate gel海藻酸钙凝胶sodium alginate海藻酸钠alginate potassium海藻酸钾alginate calcium海藻酸钙alginate lyase海藻酸裂解酶algin ink海藻酸钠墨水modified calcium alginate gel改性海藻酸钙凝胶alginate-chitosan microcapsule海藻酸钙-几丁聚糖微胶囊Ca alginate immobilized yeast海藻酸钙固定化酵母DEET-calcium alginate microsphere避蚊胺-海藻酸钙微球PVA-alginate gel PVA-海藻酸盐凝胶barium alginate microcapsule海藻酸钡微囊antibacterial alginate/ gelatin blend fiber抗菌海藻酸/明胶共混纤维poly-ornithine alginate microcapsule多聚鸟氦酸/海藻酸微囊alginate-chitosan-alginate Ion海藻酸-壳聚糖-海藻酸离子聚已酯polycaprolactone聚已酯polycarpolaction聚已酸酯 PCL ; ; polycaprolactonepolycaprolactone glycol聚已酯乙二醇poly (caprolactone)聚ε-已酯chitin-polycaprolactone bone plate甲壳素聚已酯接骨板chitin-polycaprolactone bone plate甲壳素-聚已酯接骨板poly(L-lactic acid)-block-poly(ε-caprolactone)(PLLA-b-PCL)左旋聚乳酸/聚已酯chitin-polycaprolactone bone plate甲壳素—聚已酯接骨板polycaprolactone/polyethylene glycol/polylactide (PCEL) Tri-ponent copolymer聚已酯/聚乙二醇/聚乳酸三元共聚物micropore polycaprolactone membrane微孔聚已酯膜poly(ε-caprolactone)/ poly ( ethylene glycol) block copolymer端氨基聚乙二醇-聚已酯二嵌段共聚物fibre/ polycaprolactone position竹纤维/聚已酯复合化聚羟基乙酸polyglycolic acid;PLGA聚羟基乙酸poly lactic acid-glycolic acid copolymer聚羟基乙酸共聚物poly lactic-co-glycolic acid乳酸-聚羟基乙酸polyglycolic acid scaffold聚羟基乙酸支架collagen-polyglycolic acid scaffold without cells无细胞的胶原-聚羟基乙酸支架polyglycolic acid collagen carrier聚羟基乙酸-胶原载体poly ( glycolic acid) grafted starch copolymer聚羟基乙酸接枝淀粉共聚物injectable PLGA microspheres loading estradiol注射用雌二醇聚乳酸羟基乙酸缓释微球丝素蛋白silk fibroin丝素蛋白regenerated fibroin protein再生丝素蛋白wild antheraea pernyi silk fibroin柞蚕丝素蛋白silk fibroin protein solution丝素蛋白溶液acrylic fibre silk protein丝素蛋白腈纶porous silk fibroin多孔丝素蛋白silk fibre丝素蛋白纤维PLA/丝素蛋白 PLA/silk fibroin石墨烯graphene石墨烯graphene transistor石墨烯晶体管graphite o*ide film氧化石墨烯膜chemically reduced graphene o*ide原氧化石墨烯graphene quantum dot石墨烯量子点single layer graphene层石墨烯graphene paper石墨烯纸low Pt loading graphene posite低载铂量的铂/石墨烯〔Pt/RGO〕复合材料graphene/ carbon nanotube石墨烯/碳纳米管photoluminescent graphene荧光石墨烯Ni-Fe layered double hydro*ide/ graphene镍-铁层状双氢氧化物/石墨烯titanate/ o*ide grapheme/ polyurethane posite钛酸钠/石墨烯/聚氨酯复合材料聚丙烯酰胺polyacrylamide聚丙烯酰胺polyacrylamide gel聚丙烯酰胺凝胶hydrolyzed polyacrylamide水解聚丙烯酰胺methene polyacrylamide甲叉聚丙烯酰胺potassium salt of partially hydrolyzed polyacrylamide聚丙烯酰胺钾盐amphoteric polyacrylamide两性聚丙烯酰胺polyacrylamide for medical use;medical polyacrylamide医用聚丙烯酰胺polyacrylamide aqueous solution聚丙烯酰胺水溶液low-molecular weight cationic polyacrylamide低相对分子质量阳离子聚丙烯酰胺modified polyacrylamide grouting material改性聚丙烯酰胺灌浆材料polyacrylamide gel electrophoresis聚丙烯酰胺凝胶电泳chitosan-graft-polyacrylamide 壳聚糖-聚丙烯酰胺接枝共聚物cationic-type polyacrylamide emulsion 阳离子型聚丙烯酰胺乳液聚乙烯Polyethylene 聚乙烯polyvinyl聚乙烯基polyethylene film聚乙烯膜porous polyethylene多孔聚乙烯polyethylene pipe聚乙烯管linear polyethylene 线性聚乙烯low pressure polyethylene低压聚乙烯polyvinyl resin聚乙烯树脂polyvinyl ether聚乙烯醚polythene strip聚乙烯片cellular polyethylene泡沫聚乙烯polyethylene paper聚乙烯纸chlorosulfonated polyethylene e*ternal coating氯磺化聚乙烯防腐层short-chain branched polyethylene短链支化聚乙烯high-density polythylene高密度聚乙烯ultra-high molecular weight polyethylene超高分子量聚乙烯polyethelene debris cytokine聚乙烯微粒细胞因子polyethylene wa* micropowder聚乙烯蜡微粉polythene and carbon black posite material聚乙烯炭黑复合导电材料聚四氟乙烯polytetrafluoroethylene;PTFE聚四氟乙烯teflon seal聚四氟乙烯油封epo*y teflon环氧聚四氟乙烯teflon asbestos聚四氟乙烯石棉polytetrafluoroethylene resin聚四氟乙烯树脂PTFE microporous membrane聚四氟乙烯微孔薄膜e*panded polytetrafluoroethylene mesh膨体聚四氟乙烯补片e*pansible polytetrafluoroethylene膨体聚四氟乙烯聚乙烯亚胺polyethyleneimine聚乙烯亚胺polyethyleneimine resin聚乙烯亚胺树脂polyethylene imine fractionation聚乙烯亚胺沉淀modified polyethyleneimine改性聚乙烯亚胺polyethyleneimine nanoparticles as gene delivery system聚乙烯亚胺纳米基因载体hyperbranched and linear polyethylenimine超支化及线性聚乙烯亚胺纤维蛋白fibrin纤维蛋白fibrinogen纤维蛋白原fibrin glue纤维蛋白胶plasma fibrinogen血纤维蛋白原fibrinolysin纤维蛋白溶酶fibrin adhesive纤维蛋白粘着剂fibrinopeptide纤维蛋白肽myofibrillar protein肌原纤维蛋白fibrin glue stand纤维蛋白胶支架fibrin sealant纤维蛋白封闭剂modified fibrinogen posite scaffold改进纤维蛋白原支架fibrin-targeted contrast agent纤维蛋白靶向比照剂明胶gelatin明胶gelatin sponge明胶海绵gelatin medium明胶培养基hydrolyzed gelatin水解明胶animal gelatin动物明胶photogelatin ; photographic gelatin照相明胶gelatin particle明胶微粒gelatin agar明胶琼脂 TTGA ;edible glutin食用明胶bone matri* gelatin骨基质明胶silver halide sensitized gelatin hologram卤化银明胶全息图gelatin/ chitosan posite film明胶-壳聚糖复合膜dichromated gelatin重铬酸盐明胶polyvinyl alcohol-gelatin esterified hydrogel聚乙烯醇明胶酯化水凝胶gelatin/ β-tricalcium phosphate porous posite microsphere明胶/β-磷酸三钙多孔复合微球gelatin/ hydro*yapatite posite microsphere明胶/羟基磷灰石复合物微球nano-hydro*yapatite/ chitosan-gelatin posite scaffold纳米羟基磷灰石/壳聚糖-明胶复合支架材料one side anti-static gelatine coating substrate单面涂布防静电明胶底层gelatin nanoparticle纳米明胶gelatin-network gel method明胶网络凝胶法zedoary turmeric oil gelatin microsphere莪术油明胶微球自组装肽self-assembling peptide自组装肽self-assembly peptide hydrogels自组装肽水凝胶nanofiber self-assembly peptide纳米自组装肽self-assembling peptide nanotube自组装环肽纳米管self-assembly oligopeptide自组装寡肽self-assembling peptide sequence自组装短肽序列self-assembling cyclic peptide membrane环肽自组装单层膜3D打印three-dimensional printing,3D printing 3D打印3D printer 3D打印机bioprinting生物打印bio-printer生物打印机bioprinted skin生物打印的皮肤。
自组装纳米纤维支架在神经组织工程中的应用进展
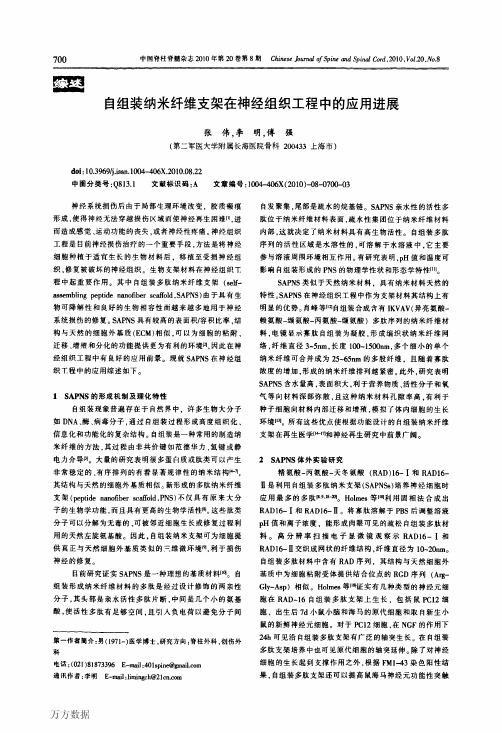
密突起穿越SAPNS治疗的损伤区域。而损伤后未经治疗
的动物损伤I)(空洞形成且无轴突再生。他们还证明了
SAPNSs在提高视束损伤再生的有效性。在上丘(SC)处横 断视束后.将SAPNSs溶液注入损伤区域。组织学结果显 示SAPNSs治疗组的动物在30、45、90d时均有穿越损伤 区域的组织再连接。治疗后90d行为学测试显示实验组
第一作者简介:男(1971一)医学博士,研究方向:脊柱外科,创伤外 科 电话:(021)81 873396
E-mail:401spine@gmail.corn
24h可见沿自组装多肽支架有广泛的轴突生长。在自组装 多肽支架培养中也可见原代细胞的轴突延伸。除了对神经 细胞的生长起到支撑作用之外,根据FMl-43染色阳性结 果,自组装多肽支架还可以提高鼠海马神经元功能性突触
assembling peptide nanofiber
自发聚集,尾部是疏水的烷基链。SAPNS亲水性的活性多
肽位于纳米纤维材料表面,疏水性集团位于纳米纤维材料 内部,这就决定了纳米材料具有高生物活性。自组装多肽 序列的活性区域是水溶性的。可溶解于水溶液中.它主要 参与溶液周围环境相互作用。有研究表明。pH值和温度可 影响自组装形成的PNS的物理学性状和形态学特性…l。 SAPNS类似于天然纳米材料。具有纳米材料天然的 特性。SAPNS在神经组织工程中作为支架材料其结构上有 明显的优势。肖峰等【・≈白组装合成含有IKVAV(异亮氨酸一 赖氨酸一缬氨酸一丙氨酸一缬氨酸)多肽序列的纳米纤维材 料,电镜显示寡肽自组装为凝胶.形成编织状纳米纤维网 络,纤维直径3—5nm。长度100—1500nto.多个细小的单个 纳米纤维可合并成为25~65nm的多股纤维,且随着寡肽 浓度的增加,形成的纳米纤维排列越紧密。此外。研究表明
Peptide-amphiphile nanofibers a versatile scaffold for the preparation of self-assembling materials
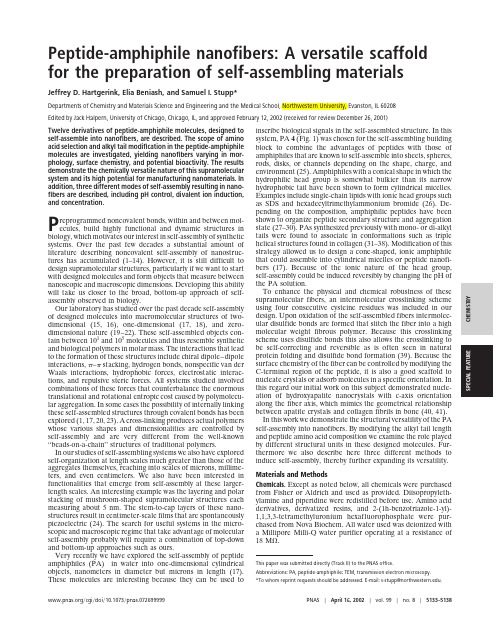
Peptide-amphiphile nanofibers:A versatile scaffold for the preparation of self-assembling materials Jeffrey D.Hartgerink,Elia Beniash,and Samuel I.Stupp*Departments of Chemistry and Materials Science and Engineering and the Medical School,Northwestern University,Evanston,IL60208Edited by Jack Halpern,University of Chicago,Chicago,IL,and approved February12,2002(received for review December26,2001)Twelve derivatives of peptide-amphiphile molecules,designed to self-assemble into nanofibers,are described.The scope of amino acid selection and alkyl tail modification in the peptide-amphiphile molecules are investigated,yielding nanofibers varying in mor-phology,surface chemistry,and potential bioactivity.The results demonstrate the chemically versatile nature of this supramolecular system and its high potential for manufacturing nanomaterials.In addition,three different modes of self-assembly resulting in nano-fibers are described,including pH control,divalent ion induction, and concentration.P reprogrammed noncovalent bonds,within and between mol-ecules,build highly functional and dynamic structures in biology,which motivates our interest in self-assembly of synthetic systems.Over the past few decades a substantial amount of literature describing noncovalent self-assembly of nanostruc-tures has accumulated(1–14).However,it is still difficult to design supramolecular structures,particularly if we want to start with designed molecules and form objects that measure between nanoscopic and macroscopic dimensions.Developing this ability will take us closer to the broad,bottom-up approach of self-assembly observed in biology.Our laboratory has studied over the past decade self-assembly of designed molecules into macromolecular structures of two-dimensional(15,16),one-dimensional(17,18),and zero-dimensional nature(19–22).These self-assembled objects con-tain between101and105molecules and thus resemble synthetic and biological polymers in molar mass.The interactions that lead to the formation of these structures include chiral dipole–dipole interactions,–stacking,hydrogen bonds,nonspecific van der Waals interactions,hydrophobic forces,electrostatic interac-tions,and repulsive steric forces.All systems studied involved combinations of these forces that counterbalance the enormous translational and rotational entropic cost caused by polymolecu-lar aggregation.In some cases the possibility of internally linking these self-assembled structures through covalent bonds has been explored(1,17,20,23).A cross-linking produces actual polymers whose various shapes and dimensionalities are controlled by self-assembly and are very different from the well-known ‘‘beads-on-a-chain’’structures of traditional polymers.In our studies of self-assembling systems we also have explored self-organization at length scales much greater than those of the aggregates themselves,reaching into scales of microns,millime-ters,and even centimeters.We also have been interested in functionalities that emerge from self-assembly at these larger-length scales.An interesting example was the layering and polar stacking of mushroom-shaped supramolecular structures each measuring about5nm.The stem-to-cap layers of these nano-structures result in centimeter-scale films that are spontaneously piezoelectric(24).The search for useful systems in the micro-scopic and macroscopic regime that take advantage of molecular self-assembly probably will require a combination of top-down and bottom-up approaches such as ours.Very recently we have explored the self-assembly of peptide amphiphiles(PA)in water into one-dimensional cylindrical objects,nanometers in diameter but microns in length(17). These molecules are interesting because they can be used to inscribe biological signals in the self-assembled structure.In this system,PA4(Fig.1)was chosen for the self-assembling building block to combine the advantages of peptides with those of amphiphiles that are known to self-assemble into sheets,spheres, rods,disks,or channels depending on the shape,charge,and environment(25).Amphiphiles with a conical shape in which the hydrophilic head group is somewhat bulkier than its narrow hydrophobic tail have been shown to form cylindrical micelles. Examples include single-chain lipids with ionic head groups such as SDS and hexadecyltrimethylammonium bromide(26).De-pending on the composition,amphiphilic peptides have been shown to organize peptide secondary structure and aggregation state(27–30).PAs synthesized previously with mono-or di-alkyl tails were found to associate in conformations such as triple helical structures found in collagen(31–38).Modification of this strategy allowed us to design a cone-shaped,ionic amphiphile that could assemble into cylindrical micelles or peptide nanofi-bers(17).Because of the ionic nature of the head group, self-assembly could be induced reversibly by changing the pH of the PA solution.To enhance the physical and chemical robustness of these supramolecular fibers,an intermolecular crosslinking scheme using four consecutive cysteine residues was included in our design.Upon oxidation of the self-assembled fibers intermolec-ular disulfide bonds are formed that stitch the fiber into a high molecular weight fibrous polymer.Because this crosslinking scheme uses disulfide bonds this also allows the crosslinking to be self-correcting and reversible as is often seen in natural protein folding and disulfide bond formation(39).Because the surface chemistry of the fiber can be controlled by modifying the C-terminal region of the peptide,it is also a good scaffold to nucleate crystals or adsorb molecules in a specific orientation.In this regard our initial work on this subject demonstrated nucle-ation of hydroxyapatite nanocrystals with c-axis orientation along the fiber axis,which mimics the geometrical relationship between apatite crystals and collagen fibrils in bone(40,41). In this work we demonstrate the structural versatility of the PA self-assembly into nanofibers.By modifying the alkyl tail length and peptide amino acid composition we examine the role played by different structural units in these designed molecules.Fur-thermore we also describe here three different methods to induce self-assembly,thereby further expanding its versatility. Materials and MethodsChemicals.Except as noted below,all chemicals were purchased from Fisher or Aldrich and used as provided.Diisopropyleth-ylamine and piperidine were redistilled before use.Amino acid derivatives,derivatized resins,and2-(1h-benzotriazole-1-yl)-1,1,3,3-tetramethyluronium hexafluorophosphate were pur-chased from Nova Biochem.All water used was deionized with a Millipore Milli-Q water purifier operating at a resistance of 18M⍀.This paper was submitted directly(Track II)to the PNAS office.Abbreviations:PA,peptide-amphiphile;TEM,transmission electron microscopy.*To whom reprint requests should be addressed.E-mail:s-stupp@.͞cgi͞doi͞10.1073͞pnas.072699999PNAS͉April16,2002͉vol.99͉no.8͉5133–5138C H E M I S T R Y S P E C I A L F E A T U R ESynthesis of the PAs.The PAs were prepared on a 0.25-mmol scaleby using standard fluorenylmethoxycarbonyl chemistry on an Applied Biosystems 733A automated peptide synthesizer.All peptides prepared have a C-terminal carboxylic acid and were made by using prederivatized Wang resin with the exception of peptide 10.In the case of peptide 10,1equivalent of Wang resin was reacted with three equivalents of fluorenylmethoxycarbonyl-Ser(PO 3Bnzl)-OH,four equivalents of 1-(mesitylene-2-sulfonyl)-3-nitro-1H-1,2,4-triazole,and six equivalents of 1-methylimidazole in dichloromethane.After the peptide por-tion of the molecule was prepared,the resin was removed from the automated synthesizer and the N terminus was capped with a fatty acid containing 6,10,16or 22carbon atoms.The alkylation reaction was accomplished by using two equivalents of the fatty acid,two equivalents 2-(1h-benzotriazole-1-yl)-1,1,3,3-tetramethyluronium hexafluorophosphate,and six equivalents of diisopropylethylamine in dimethylformamide.The reaction was allowed to proceed for at least 6h after which the reaction was monitored by ninhydrin.The alkylation reaction was re-peated until the ninhydrin test was negative.In general the longer the fatty acid the more repetitions were required to drive the reaction to completion.Cleavage and deprotection of the PAs containing cysteine was done with a mixture of triflouroacetic acid,water,triisopropyl silane,and ethanedithiol in a ratio of 91:3:3:3for 3h at room temperature.The cleavage mixture and two subsequent trif-louroacetic acid washings were filtered into a round-bottom flask.The solution was roto-evaporated to a thick viscous solution.This solution was triturated with cold diethylether.The white precipitation was collected by filtration,washed with copious cold ether,and dried under vacuum.Typically,200mg of the PA powder was dissolved in 20ml of water with the addition of 1M NaOH to adjust the pH of the solution to 8and 200mg of DTT to reduce all cysteine amino acids to the free thiol and allowed to stir overnight.The solution was then filtered through a 0.2-m nylon Acros filter into a new round-bottom flask.This 10mg ͞ml (1%by weight)solution was used for all subsequent manipulations.Work-up of PAs not containing cysteine were performed as above accept that ethanedithiol was omitted from the cleavage reaction and DTT was not used in the preparation of aqueous solutions.PAs were characterized bymatrix-assisted laser desorption ionization –time of flight MS and were found to have the expected molecular weight.Acid-Induced Self-Assembly.Samples of the PA in question wereprepared as above and placed in a small glass vial with an open top.This vial and a second vial filled with 12M HCl were placed together in a sealed glass chamber where the HCl vapor was allowed to slowly diffuse into the PA solution.Divalent Ion-Induced Self-Assembly.One hundred microliters of 10mg ͞ml solution of PA 4was treated with 1M CaCl 2(adjusted to pH 6)drop-wise in 1-l increments.The solutions were shaken after each addition of the CaCl 2solution to obtain better diffusion of the metal ions.Covalent Capture of the Assembled Fiber.The gels formed above were treated with 0.05M I 2,which was adjusted to a pH of3.5.Fig.1.Chemical structure of PA 4.Fig.2.Time sequence of pH-controlled PA self-assembly and disassembly.(Upper )From left to right molecule 6dissolved in water at a concentration of 0.5%by weight at pH 8is exposed to HCl vapor.As the acid diffused into the solution a gel phase is formed,which self-supports upon inversion (Far Left ).(Lower )The same gel is treated with NH 4OH vapor,which increases the pH and disassembles the gel,returning it to a fully dissolvedsolution.Fig.3.Light microscopy image of a gel formed from PA 4between crossed polarizers.Birefringence indicates orientation of the material at the level of tens of microns.Table 1.List of PA moleculesMoleculeN terminus Peptide (N to C)Charge pH 71HCCCCGGGS (PO 4)RGD Ϫ22C 6H 11O CCCCGGGS (PO 4)RGD Ϫ33C 10H 19O CCCCGGGS (PO 4)RGD Ϫ34C 16H 31O CCCCGGGS (PO 4)RGD Ϫ35C 22H 43O CCCCGGGS (PO 4)RGD Ϫ36C 10H 19O AAAAGGGS (PO 4)RGD Ϫ37C 16H 31O AAAAGGGS (PO 4)RGD Ϫ38C 16H 31O CCCCGGGS (PO 4)Ϫ39C 16H 31O CCCCGGGS (PO 4)KGE Ϫ310C 16H 31O CCCCGGGS (PO 4)RGDS Ϫ311C 16H 31O CCCCGGGSRGD Ϫ112C 16H 31OCCCCGGGEIKVAVϪ15134͉ ͞cgi ͞doi ͞10.1073͞pnas.072699999Hartgerink et al .The iodine solution was placed on top of the gel and allowed to slowly diffuse into the gel.After the iodine color had completely penetrated the gel,excess iodine was removed.The gel was then soaked in a bath of deionized water,which was periodically changed until the discoloration from the iodine was gone as judged by eye (roughly 48h depending on the size of the gel).The formation of the disulfide bonds was monitored by Fourier transform-IR (Fig.9,which is published as supporting informa-tion on the PNAS web site,).After oxidation the spectra revealed the effective disappearance of the S-H stretch-ing peak at 2,556cm Ϫ1.Transmission Electron Microscopy (TEM).Samples of the PAs wereprepared in two different ways.In some cases a small sample of the gel,prepared in bulk as described above,was smeared onto a holey carbon-coated TEM grid (Quantifoil,Jena,Germany).Other samples were prepared directly on the grid by placing 10l of 0.01–0.02%solution of PA directly on the grid.The grid was then placed into a sealed chamber with HCl vapors for 10min after which the grids were washed with deionized water.Two routine staining techniques,negative staining with phosphotung-stic acid or positive staining with uranyl acetate,were used in this study (42).In all cases electron microscopy was performed at an accelerating voltage of 200kV.Results and DiscussionIn an attempt to gain a better understanding of which compo-nents of the PA motif are responsible for its self-assembly properties and to explore the extent to which the molecule can tolerate modifications for use in other applications,12deriva-tives of the PA were prepared as shown in Table 1.In particular,three regions of the molecule were modified.First,the alkyl tail,which provides the hydrophobic driving force for self-assembly,was varied from as long as a 22-carbon fatty acid to molecules containing no fatty acid at all.Second,the tetra-cysteine region was replaced by a tetra-alanine repeat to determine the effects of the thiol groups on self-assembly.Finally,the head group region of the molecule (the C-terminal end),which is the segment of the molecule that interacts with the environment,was modified to incorporate different cell adhesion ligands and crystal nucleation centers.Effect of Hydrophobic Tail Length on Self-Assembly.Five differentmolecules with the peptide sequence CCCCGGGS (PO 4)RGD (molecules 1–5)were prepared and dissolved in water at a concentration between 10%and 0.001%by weight at pH 8.Uponacidification it was found that molecules 3–5formed a precipi-tate even at a concentrations as low as 0.001%by weight (0.01mg ͞ml).Molecule 2precipitated only at concentrations above 1%by weight whereas molecule 1was pH-insensitive.When a solution of molecules 3,4,or 5was slowly acidified above a concentration of 0.25%by weight the solution forms a self-supporting gel (Fig.2).The micrograph in Fig.3shows the optical texture of the gel at high concentration (12%by weight),revealing striations on the order of 10microns wide,which are reminiscent of a cholesteric liquid crystalline phase.If molecules 3–5were not fully reduced with DTT before acidification or were intentionally oxidized with I 2,subsequent acidification did not produce a gel.Instead the PA was found to precipitate as an off-white powder.Examination of the precipitate by polarized light microscopy revealed that it was not birefringent.Negative-stain TEM showed no particular supramolecular structure but rather irregular clumps with a wide size distribution.In contrast,the material precipitated from fully reduced solutions of mole-cules 3–5when investigated by negative-stain TEM were found to form a dense network of fibers in excess of 1m long and 5–8Fig.4.TEM image of fibers formed from molecules 3(a )and 5(b ).Samples were negatively stained with phosphotungsticacid.Fig.5.Schematic illustrating the self-assembly and covalent capture of the PAs based on pH and oxidation state.Molecules self-assemble upon acidi fi-cation and dissassemble at neutral and basic pH when fully reduced.Molecules that are oxidized will not self-assemble at acidic pH,likely because of the distorted conformation required by intramolecular disul fide bonds.Supramo-lecular fibers that are oxidized (polymerized)lose their sensitivity to pH and are thus stable across a much broader range of pH,including physiological.Hartgerink et al .PNAS ͉April 16,2002͉vol.99͉no.8͉5135C H E M I S T R YS P E C I A L F E A T U R Enm in diameter (Fig.4).In contrast,the precipitate formed by molecule 2formed only aggregates of random size and shape.The pH sensitivity of the different PAs can be explained as follows.When the pH of the solution is near neutrality the peptides have a net negative charge,which keeps PA molecules from self-assembly as a result of electrostatic repulsion.Upon acidification this negative charge is eliminated and hydrophobic tails can now begin to aggregate.The pH insensitivity observed in PA 1,a molecule without an alkyl tail,is a clear indication that the hydrophobic effect (43)plays a key role in self-assembly in water.Although PA 2has a hydrophobic tail,it is apparently not long enough to favor thermodynamically the extensive formation of nanofiber networks.This could be the result of geometrical effects as described by Israelachvili et al.(44)or to a weaker hydrophobic effect.However,the system is sensitive to pH,suggesting a different aggregation mechanism occurs in water after electrostatic repulsive forces are decreased.In contrast,PAs 3–5have sufficiently long hydrophobic tails to make the cylindrical packing the most favorable.However,if PAs 3–5are partially oxidized,formation of intramolecular disulfide bonds prevents the formation of nanofibers.This observation suggests that conformational changes introduced by intramolecular di-sulfide bonds suppress self-assembly.Interestingly,formation and structure of the nanofibers pre-pared from this PA was found to be unaffected by the starting concentration.However,a second level of organization,that was concentration-dependent,was revealed by TEM images.This involved the formation of flat parallel bundles containing many nanofibers.As the concentration of PA was increased the percentage of fibers involved in bundle formation increased.Given that three-dimensional networks of the high aspect ratio objects must form for gelation to occur (45),this observation would naturally explain why gelation occurs only beyond a certain threshold PA concentration of Ϸ0.25%by weight.In our previous work we showed that self-assembled nanofi-bers formed by molecule 4can be covalently captured by the formation of disulfide bonds upon oxidation with iodine (17).The same procedure was found to work for molecules 3and 5.Fig.5summarizes schematically the reversibility of both self-assembly and covalent capture in these systems.Other peptide systems have been previously reported that exhibit pH-reversible gelation (46);however,our system adds versatility to this self-assembly scaffold because of its reversible polymerization.Elimination of Crosslinking Function.We also examined here theeffect of the PA crosslinking region on self-assembling behavior.Although the cysteine residues can lead to reversible covalent capture of the nanofibers,there are some applications inwhichFig.6.TEM image of fibers formed from molecules 6(a )and 7(b ).Samples were negatively stained with phosphotungstic acid.PA 6displays a strong tendency to form parallel arrays of fibers whereas PA 7doesnot.Fig.7.TEM images of fibers formed from molecules 9(a ),11(b ),and 12(c ).Samples were negatively stained with phosphotungstic acid.Although fibers with a diameter between 5and 8nm are formed in all cases,the length and stiffness of the fibers formed vary considerably.5136͉ ͞cgi ͞doi ͞10.1073͞pnas.072699999Hartgerink et al .the presence of thiols can be problematic,such as catalysis.For example,transition metal catalysts can be poisoned by sulfur (47).Furthermore,the cysteines must be fully reduced for self-assembly to be successful,which requires that this be done in the presence of DTT or in an anaerobic environment.To eliminate this problem,the cysteine amino acids were replaced by alanine.Two derivatives were prepared,one with a C10alkyl tail (molecule 6)and one with a C16alkyl tail (molecule 7).Self-assembly was accomplished by gas-phase acidification as before and in both cases a self-supporting gel was formed at concentrations of 0.2%and above.Examination of the gels by negative-stain TEM revealed that the gels are made up of nanofibers with similar structure compared with the cysteine-containing nanofibers.This finding indicates the presence of cysteine residues is not required for self-assembly.However,in the case of molecule 6the propensity for packing into broad ribbons of fibers appears to be much higher (Fig.6a ).In contrast,molecule 7,although maintaining the same fiber superstructure,appears to form almost no parallel arrangements between fibers (Fig.6b ).Additionally,this gel when examined after several days exhibits some degree of phase separation.Even though it is not clear at this point why this occurs,it may well be an indication of a transition from a kinetic gel to a more thermodynamically stable state.Versatility of the Hydrophilic C-Terminal Region.The third region ofthe PA investigated for modification is the head group of the molecule,which is exposed on the surface of nanofibers after self-assembly.It is this portion of the molecule that will interact directly with the environment and may be modified to enhance bioactivity,control mineralization of inorganic struc-tures,promote catalysis,or gain other desirable properties.PAs 8–12were prepared to establish whether this region could be altered while still maintaining a fibrous morphology.Mol-ecules 8–10and 12were prepared to examine the ability to display chemistries with different cell adhesion properties.In the original design of the PAs we included phosphoserine to control mineralization (17).In this work we synthesized de-rivatives 11and 12to determine whether phosphorylation is required for self-assembly.In one case,molecule 12,we used the sequence IKVAV because of its known role as a cell adhesion ligand in laminin (48,49).This molecule differs from the original PA,which contains the integrin binding sequence RGD,because it has significant hydrophobic content and alsolacks a phosphate group.PA 8,which does not contain a bioactive sequence,was also found to form nanofibers.At the same time,PAs 9and 10,which contain control sequences for bioactivity experiments,were also found to exhibit self-assembling behavior.Interestingly,despite chemical differ-ences,the general design of our self-assembling scaffold is conducive to the formation of one-dimensional nano-structures (Fig.7).However,our TEM observations show that fibers differ in length and stiffness.For example PA 6fibers are longer than PA 7(Fig.6),whereas fibers formed by PA 12appear to be stiffer that ones formed by PA 11.Different Modes of Self-Assembly.Finally,we also explored al-ternative modes of promoting self-assembly that do not require pH changes.These alternative modes may be of interest for the use of these systems in medicine.The pH-triggered mechanism we used is a powerful method to control the state of aggre-gation,but it also restricts the noncrosslinked supramolecular structures to particular pH regimes.First,we found that self-assembly can occur by simply taking a PA dissolved in water at pH 8and placing it on a surface that is allowed to dry (for example,directly on a carbon-coated TEM grid).Upon examination of this preparation by negative-stain TEM we clearly observed the formation of nanofibers (Fig.8a ).We are not sure at the moment what the mechanism is that drives self-assembly at this high pH;however,three important factors are likely to be charge screening by the surface and increased concentration of salts and PA as the water evaporates.We have also found that treatment of a solution of PA 4with a divalent ion,such as Ca 2ϩ,immediately causes gelation of the solution.In contrast,treatment of PA samples with K ϩup to 6M does not lead to gelation or self-assembly.When examined by positive-stain TEM the gel formed with calcium ions was found to be composed of nanofibers with the same dimensions as those formed by acid-induced self-assembly and by drying on surfaces.This calcium-induced self-assembly may be particu-larly useful for medical applications where formation of a gel at physiological pH is desired.ConclusionsA family of PAs has been demonstrated to self-assemble revers-ibly into nanofiber networks,which result in the formation of aqueous gels through pH changes.The PA fibers can then be reversibly polymerized to enhance their stability.ThesetwoFig.8.TEM images of molecule 3(a )self-assembled by drying directly onto a TEM grid without adjusted pH and molecule 4(b )self-assembled by mixing with CaCl 2.Molecule 3is negatively stained with phosphotungstic acid whereas molecule 4is positively stained with uranyl acetate.In both cases the same fibrous morophology is observed as is seen by pH-induced self-assembly.Hartgerink et al .PNAS ͉April 16,2002͉vol.99͉no.8͉5137C H E M I S T R YS P E C I A L F E A T U R Eswitchable events controlling the formation of supramolecular structure and polymerization produce a remarkably versatile material.This versatility has been expanded here to include two methods of self-assembly,drying on surfaces and the addition of divalent ions such as calcium.Twelve variants of the PA,with changes in alkyl tail length,the polymerizable region,and the C-terminal peptide sequence all have been shown to self-assemble into a one-dimensional fibrous motif.These molecules demonstrate the ability of the system to tolerate chemi-cal modification for use in both biological and nonbiological applications.We thank Bryan Rabatic for assistance with matrix-assisted laser de-sorption ionization–time of flight MS and the EPIC center at North-western University for use of its Hitachi H8100transmission electron microscope.This work was supported by the Department of Energy (Grant DE-FG02-00ER45810͞A001)and the Air Force Office of Sci-entific Research(Grant F49620-00-1-0283͞P01).1.Clark,T.D.,Kobayashi,K.&Ghadiri,M.R.(1999)Chem.Eur.J.5,782–792.2.Lehn,J.M.(1995)Supramolecular Chemistry:Concepts and Perspectives(VCH,Weinheim,Germany).3.Hartgerink,J.D.,Clark,T.D.&Ghadiri,M.R.(1998)Chem.Eur.J.4,1367–1372.4.Atwood,J.L.,Lehn,J.M.,Davies,J.E.D.,MacNicol,D.D.&Vogtle,F.(1996)Comprehensive Supramolecular Chemistry(Pergamon,New York).5.Ghadiri,M.R.,Granja,J.R.,Milligan,R.A.,McRee,D.E.&Khazanovich,N.(1993)Nature(London)366,324–327.6.Brunsveld,L.,Folmer,B.J.B.&Meijer,E.W.(2000)MRS Bull.25,49–53.7.Hirschberg,J.H.K.K.,Brunsveld,L.,Ramzi,A.,Vekemans,J.A.J.M.,Sijbesma,R.P.&Meijer,E.W.(2000)Nature(London)407,167–170.8.Engelkamp,H.,Middelbeek,S.&Nolte,R.J.M.(1999)Science284,785–788.9.Isaacs,L.,Chin,D.N.,Bowden,N.,Xia,Y.&Whitesides,G.M.(1999)Perspect.Supramol.Chem.4,1–46.10.Whitesides,G.M.,Mathias,J.P.&Seto,C.T.(1991)Science254,1312–1319.11.MacDonald,J.C.&Whitesides,G.M.(1994)Chem.Rev94,2383–2420.12.Hudson,S.D.,Jung,H.-T.,Percec,V.,Cho,W.-D.,Johansson,G.,Ungar,G.&Balagurusamy,V.S.K.(1997)Science278,449–452.13.Percec,V.,Ahn,C.-H.,Ungar,G.,Yeardley,D.J.P.,Moller,M.&Sheiko,S.S.(1998)Nature(London)391,161–164.14.Zimmerman,S.C.,Zeng,F.,Reichert,D.E.C.&Kolotuchin,S.V.(1996)Science271,1095–1098.15.Stupp,S.I.,Son,S.,Lin,H.C.&Li,L.S.(1993)Science259,59–63.16.Stupp,S.I.,Son,S.,Li,L.S.,Lin,H.C.&Keser,M.(1995)J.Am.Chem.Soc.117,5212–5227.17.Hartgerink,J.D.,Beniash,E.&Stupp,S.I.(2001)Science294,1684–1688.18.Zubarev,E.R.,Pralle,M.U.,Sone,E.D.&Stupp,S.I.(2001)J.Am.Chem.Soc.123,4105–4106.19.Stupp,S.I.,LeBonheur,V.,Walker,K.,Li,L.S.,Huggins,K.E.,Keser,M.&Amstutz,A.(1997)Science276,384–389.20.Zubarev,E.R.,Pralle,M.U.,Li,L.&Stupp,S.I.(1999)Science283,523–526.21.Tew,G.N.,Pralle,M.U.&Stupp,S.I.(2000)Angew.Chem.Int.Ed.39,517–521.22.Radzilowski,L.H.&Stupp,S.I.(1994)Macromolecules27,7747–7753.23.Won,Y.Y.,Davis,H.T.&Bates,F.S.(1999)Science283,960–963.24.Pralle,M.U.,Urayama,K.,Tew,G.N.,Neher,D.,Wegner,G.&Stupp,S.I.(2000)Angew.Chem.Int.Ed.39,1486–1489.25.Israelachvili,J.N.,Mitchell,D.J.&Ninham,B.W.(1977)Biochim.Biophys.Acta470,185–201.26.Israelachvili,J.N.(1992)Intermolecular and Surface Forces(Academic,Lon-don).27.Klok,H.A.,Langenwalter,J.F.&Lecommandoux,S.(2000)Macromolecules33,7819–7826.28.Kogiso,M.,Okada,Y.,Hanada,T.,Yase,K.&Shimizu,T.(2000)Biochim.Biophys.Acta1475,346–352.29.Gore,T.,Dori,Y.,Talmon,Y.,Tirrell,M.&Bianco-Peled,H.(2001)Langmuir17,5352–5360.30.Hu,J.C.,O’Shea,E.K.,Kim,P.S.&Sauer,R.T.(1990)Science250,1400–1403.31.Berndt,P.,Fields,G.B.&Tirrell,M.(1995)J.Am.Chem.Soc.117,9515–9522.32.Fields,G.B.,Lauer,J.L.,Dori,Y.,Forns,P.,Yu,Y.-C.&Tirrell,M.(1998)Biopolymers47,143–151.33.Haverstick,K.,Pakalns,T.,Yu,Y.-C.,Mccarthy,J.B.,Fields,G.B.&Tirrell,M.(1997)Polym.Mater.Sci.Eng.77,584–585.34.Pakalns,T.,Haverstick,K.L.,Fields,G.B.,McCarthy,J.B.,Mooradian,D.L.&Tirrell,M.(1999)Biomaterials20,2265–2279.35.Yu,Y.-C.,Berndt,P.,Tirrell,M.&Fields,G.B.(1996)J.Am.Chem.Soc.118,12515–12520.36.Yu,Y.-C.,Pakalns,T.,Dori,Y.,Mccarthy,J.B.,Tirrell,M.&Fields,G.B.(1997)Methods Enzymol.289,571–587.37.Yu,Y.-C.,Tirrell,M.&Fields,G.B.(1998)J.Am.Chem.Soc.120,9979–9987.38.Yu,Y.-C.,Roontga,V.,Daragan,V.A.,Mayo,K.H.,Tirrell,M.&Fields,G.B.(1999)Biochemistry38,1659–1668.39.Anfinsen,C.B.(1973)Science181,223–230.40.Traub,W.&Weiner,S.(1989)A86,9822–9826.41.Weiner,S.&Wagner,H.D.(1998)Annu.Rev.Mater.Sci.28,271–298.42.Harris,J.R.(1991)in The Practical Approach Series,eds.Rickwood,D.&Hames,B.D.(Oxford Univ.Press,New York),p.308.43.Privalov,P.L.&Gills,S.J.(1989)Pure Appl.Chem.61,1097–1104.44.Israelachvili,J.N.,Mitchell,D.J.&Ninham,B.W.(1977)Biochem.Biophys.Acta470,185–201.45.Djabourov,M.(1991)Polym.Int.25,135–143.46.Petka,W.A.,Hardin,J.L.,McGrath,K.P.,Wirtz,D.&Tirrell,D.A.(1998)Science281,389–392.47.Rodriguez,J.A.&Hrbek,J.(1999)Acc.Chem.Res.32,719–728.48.Thompson,H.L.,Burbelo,P.D.,Yamada,Y.,Kleinman,H.K.&Metcalfe,D.D.(1991)Immunology72,144–149.49.Tashiro,K.,Sephel,G.C.,Weeks,B.,Sasaki,M.,Martin,G.R.,Kleinman,H.K.&Yamada,Y.(1989)J.Biol.Chem.264,16174–16182.5138͉͞cgi͞doi͞10.1073͞pnas.072699999Hartgerink et al.。
肽类小分子自组装分子设计、结构调控与功能化应用

摘要本课题以肽类小分子为研究对象,设计新型自组装三肽,构建其手性自组装体系,设计氨基酸衍生物的酶促组装体系和“多糖-二肽”共组装体系,调控组装过程,剖析组装机理,合成具有特定结构、功能的肽基组装材料。
(1)设计了两个新型三肽分子:Fmoc-Phe-Trp-Lys-OH(Fmoc-FWK)和Fmoc-Phe-Trp-Lys-NH2(Fmoc-FWK-NH2)。
Fmoc-FWK具有手性自组装特性,能够自组装形成具有左手螺旋结构的纳米带,而Fmoc-FWK-NH2则自组装形成不具有螺旋结构的纳米纤维。
末端羧基在螺旋结构的形成中起着关键作用,螺旋结构的稳定需要较强的π-π相互作用和静电相互作用。
(2)Fmoc-FWK手性自组装的精确调控与机理剖析。
Fmoc-FWK分子末端的电荷能够驱动其自组装形成螺旋β-折叠片,从而进一步组装形成具有特定形貌的手性纳米材料。
通过改变溶剂、pH和温度等条件能够调控β-折叠片扭转的程度和方向,从而获得螺距、宽度和手性可控的手性纳米材料。
(3)基于酶的疏水性空腔,构建氨基酸衍生物的酶促组装体系,合成超分子水凝胶。
α-胰凝乳蛋白酶能够促进疏水性Fmoc-氨基酸(如Fmoc-F,Fmoc-W)和氨基酸酯(如F-OMe,F-OEt、Y-OMe)自组装形成水凝胶,根据氨基酸结构的不同,将其成胶时间从8天(或2周内不能形成水凝胶)缩短到10 min-4 h。
在组装过程中,氨基酸酯被水解成氨基酸,Fmoc-氨基酸和氨基酸发生了共组装。
酶-底物相互作用在α-胰凝乳蛋白酶促进氨基酸衍生物自组装形成水凝胶过程中发挥着关键作用。
(4)构建Fmoc-FF与魔芋葡甘聚糖(KGM)共组装体系,制备Fmoc-FF/KGM 复合气凝胶。
以Fmoc-FF和KGM为原料,通过pH调节和溶剂稀释两种方法调控Fmoc-FF与KGM共组装形成Fmoc-FF/KGM复合水凝胶,进一步运用冷冻干燥技术制备了Fmoc-FF/KGM复合气凝胶。
Self-assembly of Peptide-Amphiphile C12-A(11-17) into Nanofibrils

Self-assembly of Peptide-Amphiphile C12-A (11-17)into NanofibrilsManli Deng,Defeng Yu,Yanbo Hou,and Yilin Wang*Beijing National Laboratory for Molecular Sciences,Key Laboratory of Colloid and Interface Science,Institute of Chemistry,Chinese Academy of Sciences,Beijing100190,People’s Republic of ChinaRecei V ed:April22,2009A peptide-amphiphile(C12-A (11-17))was constructed with a key fragment of amyloid -peptide(A (11-17))attached to dodecanoic acid through an amide bond.The self-assembly behavior ofC12-A (11-17)in aqueous solution is studied at25°C and at pH3.0and10.0.A (11-17)cannot formordered self-assemblies.But C12-A (11-17)exhibits a very strong ability to form ordered nanofibrils,andthe specificfine structure of the nanofibrils can be modulated simply by adjusting the concentration or pH.The critical micelle concentration of C12-A (11-17)was determined as0.063and0.11mM at pH3.0and10.0,respectively,indicating a stronger assembling ability of C12-A (11-17)at acidic pH.In0.47mMC12-A (11-17)solution at pH3.0,rodlikefibrils with a diameter of∼5nm and varying length of hundredsof nanometers are observed.When the C12-A (11-17)concentration increases to1.87mM at pH3.0,theabove rodlikefibrils pack in parallel and form tapelikefibrils through lateral association.In1.87mMC12-A (11-17)solution at pH10.0,twistedfibrils with regular periodicity of∼200nm are formed by thetwisting of∼20nm wide and∼11nm thick nanoribbons.The hydrophobic moiety is necessary infibrilformation,whereas the -sheet secondary structure of the peptide moiety plays an essential role in the twistingmorphology.This work helps to understand the possible mechanism in amyloidfibrillogenesis and providesan approach to inscribe biological signals in self-assemblies with potential application in biomaterial fabrication.IntroductionSelf-assembly of peptide-based amphiphiles have recently emerged as one of the most promising scaffolding for nano-/ microscale biomaterials in tissue engineering1-3and other medical applications such as regenerative medicine4,5and controlled drug release.6Various classes of peptide-based amphiphileshavebeenreported,includingamphiphilicpeptides7-10 and peptide-amphiphiles.11-14Amphiphilic peptides are usually constructed with hydrophobic and hydrophilic amino acid residues,including the structures of a charged head attached to anonpolartail,15-17alternatingpositive/negative6,9orpolar/nonpolar7,18,19 amino acid repeat units,and well-designed R-helix with hy-drophilic and hydrophobic faces.20,21These chemical sequences lead to aggregates of different hierarchies ranging from vesicle to nanotubes,and from twisted ribbons tofibril networks. Peptide-amphiphile is a kind of synthetic surfactant that typically contains one or more long alkyl chains attached to a peptide moiety.It has been found that many peptide-amphiphiles assemble into nanofibers in aqueous solution,structurally similar to cylindrical micelles,in which the alkyl tails bury in the core of thefibers while the hydrophilic oligopeptides pack on the fiber’s surface.The interactions that lead to the formation of these structures include chiral dipole-dipole interaction,π-πstacking,hydrogen bond,nonspecific van der Waals interaction, hydrophobic force,electrostatic interaction,and repulsive steric force.All systems studied involved combinations of these forces that counterbalance the enormous translational and rotational entropic cost caused by poly molecular aggregation.22Particu-larly,the -sheet formation between the peptide regions of peptide-amphiphile molecules has been recognized as a predominant factor in directing the self-assembly into cylindrical architecture as opposed to spherical micelles or vesicles.15,23,24 Amyloid -peptide(A ),a39-43-residue amphiphilic pep-tide,is the major component of the neuritic plaques found in the brains of patients suffering from Alzheimer’s disease.The secondary structure conversion from random coil or R-helix to -sheet conformation has been proposed as one of the critical reasons for the peptidefibrillogenesis and neurotoxicity.25Earlier studies26,27have demonstrated that the trifluoroethanol-stabilized monomeric A (1-42)delineated two separated helical domains, but only the destabilization of helix I,comprising residues 11-24,caused a transition to a -sheet structure and a consequent amyloid formation.Therein,the hydrophobic region between positions17and21makes up the -sheet core of the amyloidfibrils,28,29and A (16-22)is the smallest fragment of A known to formfibrils.8,30The most stable helical region is located between positions11and17,27which significantly contributes to the stability of the R-helical secondary structure of A and the inhibition of peptidefibrillogenesis. Herein,the A fragment of residues11-17(EVHHQKL)is taken as the head group of a peptide-amphiphile.The introduc-tion of A (11-17)endows the desired molecule with biological features,while the alkyl chain attached onto the N-terminal of A (11-17),serving as the hydrophobic region17-21of A , facilitates the molecular assembly.The chemical structure and space-filling model of the designed peptide-amphiphile (C12-A (11-17))are shown in Figure1.The pH and concen-tration dependence of C12-A (11-17)self-assembly in aqueous solution are investigated by isothermal titration microcalorim-etry, -potential,circular dichroism,atomic force microscopy, and transmission electron microscopy.*To whom correspondence should be addressed.E-mail:yilinwang@.J.Phys.Chem.B2009,113,8539–8544853910.1021/jp904289y CCC:$40.75 2009American Chemical SocietyPublished on Web06/01/2009Experimental SectionMaterial.The heptapeptide A (11-17)was purchased from GL Biochem (Shanghai)Ltd.We designed peptide -amphiphile C 12-A (11-17)and its synthesis was carried out by GL Biochem (Shanghai)Ltd.The purities of A (11-17)and C 12-A (11-17)were checked by high-performance liquid chromatography (better than 98%).The inorganic reagents (>99.5%)were purchased from Beijing Chemical li-Q water (18M Ω·cm -1)was used throughout.Sample Preparation.An appropriate amount of C 12-A (11-17),with a molecular weight of 1072.30,was dissolved in water to obtain the desired concentration,and then the solution was vortex-mixed.The pH was adjusted with concentrated HCl or NaOH solution to achieve the desired values.Isothermal Titration Microcalorimetry (ITC).The calo-rimetric measurements were taken in a TAM 2277-201micro-calorimetric system (Thermometric AB,Ja ¨rfa ¨lla,Sweden)with a 1mL stainless steel sample cell at 25.00(0.01°C.The cell was initially loaded with 0.6mL of water of pH 3or 10.Each aliquot of 8µL of concentrated C 12-A (11-17)solution at the corresponding pH was injected consecutively into the stirred sample cell via a 500µL Hamilton syringe controlled by a 612Thermometric Lund pump until the desired concentration range had been covered.The interval between two injections was 10min,which was long enough for the signal to return to the baseline.The observed enthalpy (∆H obs )was obtained by integrating the areas of the peaks in the plot of thermal power against time.-Potential Measurements.The -potential measurements were performed at 25.0(0.1°C using a Malvern Zetasizer Nano-ZS instrument (ZEN3600,Malvern Instruments,Worces-tershire,UK)equipped with a 4mW He -Ne laser at a wavelength of 633nm.Circular Dichroism.Circular dichroism (CD)spectra were recorded on a JASCO J-815spectrophotometer at room tem-perature using a 0.1mm quartz cell.Scans were obtained in a range between 190and 260nm by taking points at 0.5nm,with an integration time of 0.5s.Five spectra were averaged to improve the signal-to-noise ratio and smoothed using the noise-reducing option in the software supplied by the vendor.The results were represented as machine unit (θ,in units of mdeg).X-ray Diffraction.Self-supported cast films were prepared by dispersing the aqueous solutions of C 12-A (11-17)onto precleaned silicon wafers and then dried under vacuum.Reflec-tion X-ray diffraction (XRD)studies were carried out with an X-ray diffractometer (XRD-7000,Shimadzu,Japan).The X-ray beam was generated with a Cu anode at 40kV and 30mA,and the wavelength of the K R 1beam was 1.5406Å.The X-ray beamwas directed to the edge of the film,and the scanning 2θwas recorded from 1to 15°,using a step width of 0.02°.Atomic Force Microscopy.For ambient atomic force microscopy (AFM)imaging,10µL of C 12-A (11-17)solution was deposited onto a freshly cleaved piece of mica and left to adhere for 10min.The sample was then rinsed with 100µL of water and dried with a gentle stream of nitrogen.AFM imaging was performed at room temperature using the tapping mode on a Multimode Nanoscope IIIa AFM (Digital Instruments,CA).Probes used are etched silicon probes with a typical tip radius of ∼10nm,attached to 125µm cantilevers with a nominal spring constant of 40N/m (Digital Instruments,model RTESPW).All images were acquired with a scan speed of 1.0-1.5Hz,a tip resonance frequency of 230-300kHz,and a drive amplitude of 20-100mV.Height data were collected as 512×512pixel images.Topographic data were regularly recorded in both trace and retrace to check on scan artifacts.Analysis of the images was carried out using the Digital Instruments Nanoscope software (version 512r2).In each case,at least 50individual fibrils from different samples were analyzed at different parts.Transmission Electron Microscopy.Transmission electron microscopy (TEM)samples were prepared from C 12-A (11-17)solutions using negative-staining method,and 1%uranyl acetate solution was used as the staining agent.A drop of the solution was placed onto a carbon Formvar-coated copper grid,and the excess liquid was sucked away by filter paper.After drying,the samples were imaged under an electron microscope (FEI Tecnai 20).Results and DiscussionSince peptide -amphiphile C 12-A (11-17)is pH-sensitive,two pHs,3.0and 10.0,were selected to define the protonation states of the C 12-A (11-17)molecules in aqueous solution.The p K a values of the carboxylic group in Leu and in the side chain of Glu,the imidazolyl group in His,and the p K b value of the amino group in Lys are 2.36, 4.25, 6.00,and 10.53,respectively.13Thus,the peptide -amphiphile molecule carries approximately two positive net charges at pH 3.0but two negative net charges at pH 10.0.This can be verified by the comparable absolute values of the -potential values for the 1.87mM C 12-A (11-17)solution at the two pHs,as presented in Table 1.ITC measurements were conducted at 25.00°C to determine the critical micelle concentrations (CMC)of C 12-A (11-17)at pH 3.0and 10.0,respectively.Actually,the self-assemblies of C 12-A (11-17)in aqueous solution have irregular micellar morphology,referring to the following part of the discussion.The present CMC is used to describe the formation of any structure with micellar characteristics.The calorimetric titration curves of the observed enthalpy (∆H obs )are plotted against the final C 12-A (11-17)concentration (C )in Figure 2.The CMC can be identified as the extreme of the differentiating observed enthalpy curve with respect to C .The apparent enthalpy change of micellization (∆H mic )is obtained from the enthalpy difference between the two linear segments of the enthalpy curve extrapo-lated to the CMC (Table 1).The slightly smaller CMC in the acidic solution indicates the stronger assembling abilityofFigure1.Chemicalstructureandspace-fillingmodelofpeptide -amphiphile C 12-A (11-17),with a peptide sequence of EVHHQKL from N-to C-terminal.TABLE 1:Values of CMC,∆H mic ,and -Potential for the C 12-A (11-17)Solution of pH 3.0and pH 10.0at 25.0°CCMC (mM)∆H mic (kJ/mol)-potential (mV)pH 3.00.06313.5149.8pH10.00.1095.08-54.28540J.Phys.Chem.B,Vol.113,No.25,2009Deng et al.C 12-A (11-17)at pH 3.0.The ∆H mic is endothermic at both acidic and alkaline conditions,suggesting the micellization is an entropy-driven process.The endothermic ∆H mic should be mainly contributed from the electrostatic repulsion between the C 12-A (11-17)molecules with either positive or negative charges as well as the dehydration effect accompanied by the formation of the hydrogen bond between the amino acid residues.In addition,the much less endothermic ∆H mic at pH 10.0implies that the corresponding micellization process may involve less strong intermolecular repulsions with respect to that at pH 3.0.To characterize the secondary structure of the C 12-A (11-17)assemblies,CD measurements were performed at room temperature for 1.87mM C 12-A (11-17)solutions at pH 3.0and 10.0and the A (11-17)solutions at the same conditions (Figure 3).The 2.2mM A (11-17)solutions at pH 3.0and 10.0were both found to be a random coil secondary structure signaled by the minimum around 198nm.That is to say,the pH does not affect the secondary structure of A (11-17).In the case of 1.87mM C 12-A (11-17),the self-assemblies formed at pH 3.0inherit the random coil secondary structure of the peptide sequence,but at pH 10.0,the self-assemblies display a typical -sheet secondary structure as a single minimum at approximately 220nm.These results suggest both the introduction of the C 12alkyl chain and the change in pH affect the peptide secondary structure.At pH 3.0,the C 12-A (11-17)molecules could assemble through hydropho-bic interaction at the concentration used,but the two positively charged His residues in the middle of the head group enhance the electrostatic repulsion to prevent the formation of ordered secondary structure.At pH 10.0,besides the hydrophobic interaction among the alkyl chains,the disappearance ofrepulsion between intermolecular His residues facilitates the hydrogen bonding within the whole peptide moiety,which induces the formation of the -sheet secondary structure.This preference has also been suggested by the value of ∆H mic at these two pH conditions.The difference in the secondary structure of the peptide moiety at different pHs may play a crucial role in the assembly morphology of the peptide amphiphile.To identify the morphol-ogy of the C 12-A (11-17)self-assemblies,complementary AFM and TEM were carried out on its aqueous solutions at different concentrations above CMC and at different pHs.The corresponding heptapeptide fragment A (11-17)was first studied for comparison.All the sample solutions were incubated at 25.0(0.5°C for at least 24h before imaging to achieve the stable assembly states.As shown in Figure 4,only small spherical self-assemblies are observed for 2.2mM A (11-17)at both pHs.The heights of these self-assemblies range from 1to 5nm,which should be the peptide oligomers.32The morphology remains unchanged for more than 3weeks after the solution preparation.That A (11-17)could not organize into ordered assemblies may be attributed to its lack of hydrophobic interaction and the consequent weak self-assembly ability.Figure 5presents the morphologies of the C 12-A (11-17)assemblies formed at 0.47and 1.87mM,at pH 3.0and 10.0,respectively.The assemblies exhibit fibril morphology at all the conditions,but vary remarkably in fine structures depending on both concentration and pH.In 0.47mM C 12-A (11-17)solution at pH 3.0,rodlike nanofibrils are observed in the AFM image (Figure 5A 2),with an average height of 2.5(0.5nm and varying length from about 50to 200nm.The height value is very close to the calculated length of a C 12-A (11-17)molecule,so the observed assembles on mica is possibly the collapsed C 12-A (11-17)cylindrical micelles.Meanwhile,the fibrils observed in TEM image (Figure 5A 1)are a little largerinFigure 2.Variation of the observed enthalpy (∆H obs )with the final C 12-A (11-17)concentration (C )at 25.00(0.01°C and at pH 3.0and10.0.Figure 3.CD spectra of A (11-17)and C 12-A (11-17)solutions at pH 3.0and 10.0.Self-assembly of Peptide -Amphiphile C 12-A (11-17)J.Phys.Chem.B,Vol.113,No.25,20098541dimension with a 10-30nm width and 100-400nm length.And it can be seen from the terminals of these fibrils that each one is composed of two or more cylindrical micelles by bundling together.The difference in morphology between AFM and TEM images may be from the drying process.The AFM sample was rinsed with water before drying;thus,the assemblies were highly dispersed.Contrarily,there was condensation during drying of the TEM sample in which the assemblies tended to aggregate.This effect is particularly remarkable for the assemblies in small size,which has a relatively high diffusion coefficient in aqueous solution and is very sensitive to the concentration.Compared with the situation of A (11-17),the primary driven force of the C 12-A (11-17)self-assembly should be hydrophobic interaction between the alkyl chains of the peptide -amphiphile molecules.The cylindrical micelle of C 12-A (11-17)should be constructed by the hydrophobic alkyl tails emerging into the core region and leaving the hydrophilic peptide moieties exposed to water.But the high aspect ratio of the micelle geometry should be attributed to the hydrogen bonds between the peptide moieties.The importance of hydrogen bonds in the formation of nanofibrils has been recognized and emphasized in the literature.12,19,33A systematic study 11has been performed on a series of peptide -amphiphiles with a seven-glycine linker region localized between an alkyl tail and a four-residue head group.It was pointed out that the formation of the hydrogen bonds close to the hydrophobic alkyl tails is the necessary controlling factor in the formation of cylindrical shape.In the present system,the assembled alkyl chains could generate the possibilities of intermolecular hydrogen bonds between the nearby amino acid residues (such as Glu and Val),which may lead to the observed cylindrical geometry of the self-assemblies,though no -sheet structure formed.To clarify the concentration dependence of the assembly morphology,TEM and AFM measurements were also performed on 1.87mM C 12-A (11-17)solution at the same pH,i.e.,3.0.Quite long fibrils,up to micrometers,are observed (Figure5B).Figure 4.Morphologies of 2.2mM A (11-17)at (A)pH 3.0and (B)pH 10.0.Both of the images are 2×2µm 2in size and 5nm inheight.Figure 5.From left to right are the TEM images,AFM height images,and section analyses of the C 12-A (11-17)assemblies at different concentrations and pHs:(A)0.47mM,pH 3.0;(B)1.87mM,pH 3.0;and (C)1.87mM,pH 10.0.All the AFM images are 2×2µm 2in size,and the scale bars represent 200nm in all TEM images.8542J.Phys.Chem.B,Vol.113,No.25,2009Deng etal.As clearly seen from Figure5B2,the longfibrils are coexisting with shortfibrils in the background,identical to that formed at 0.47mM.It implies that the increase in the micelle’s concentra-tion may drive the C12-A (11-17)cylindrical micelles to grow from low-order(short)fibrils into high-order(long)fibrils through hydrogen bonding in larger scale.Besides,it is noted that high-orderfibrils,with length of hundreds of nanometers, consecutively adsorbed on the substrate(in the middle of the AFM image),signaling the rigid nature of thefibrils formed at pH3.0.However,the major distinction between the high-order and low-orderfibrils is the height,whose value of the former is at least2times that of the latter.Thus,the high-orderfibrils might be assembled from the elongated cylindrical C12-A (11-17)micelles without breakdown on mica.Two predominant morphologies of the high-orderfibrils are observed from both TEM and AFM images,thefilaments of5-15nm in width and the nanotapes above50nm in width.Both of them should be constructed by the lateral association of the cylindricalmicelles in varying numbers,as occurred in the amyloid fibrillogenesis.32Because the micelle surface is covered by three residues(Gln,Lys,and Leu)at the C-terminal,which are nonionic at pH3.0and are prone to form hydrogen bonds not only in the backbones but also in the side chains,the micelles could draw close to each other and bind through hydrogen bonds.That is to say,the high-orderfibrils are fabricated by a hydrogen bond network,which plays a crucial role in the assembly geometry and in further association of the primary assemblies.To know the pH dependence of the assembly morphology, TEM and AFM measurements were then performed on the1.87 mM C12-A (11-17)solution at pH10.0.Distinctive morphol-ogy of thefibrils are seen(Figure5C).Different from thefibrils at pH3.0,all thefibrils at pH10.0are in a regular twisting conformation and some of them entwine with each other.The handedness of thefibrils seems random,either left-handed or right-handed within each individual.Oda et al.34discriminated the twisting assemblies into helical ribbons with a cylindrical curvature from twisted ribbons with a Gaussian or saddlelike curvature.In the present case,fibrils in various twisting conformations coexist(Figure5C1),and the twisting ribbons should be the predominant category.Baumann et al.35revealed that,for short amphiphilic peptides, -sheet secondary structure correlates with ribbonlike assemblies while random coil structure correlates with rodlike assemblies.Here,it could be assumed that the ribbon is constructed by the -sheet secondary structure of the C12-A (11-17)peptide moiety.The peptide backbones form intermolecular hydrogen bonds perpendicular to the ribbon’s long axis.And each -stand would pack with the next one along the ribbon’s long axis in a small twisting angle as a result of the steric hindrance of the amino acid side chains(such as the imidazolyl group),which cause the ribbon to twist.From the TEM image,the average values of the thickness,the width, and the twisting periodicity of the twisting ribbon were measured as12(1,19(1,and200(10nm,respectively.Of particular, the AFM image(Figure5C2)shows perfect twisting periodicity for most of thefibrils,as obviously seen from the section analysis in profile along thefibril long axis.The periodicity can be measured from the horizontal distance of the two neighboring peaks or the average of a number of the peaks,as marked by the black arrows.Because the magnitude of the periodicity is far larger than the assemble height,the AFM tip-related convolution effect could be neglected.The mean value is obtained as190(15nm,in good agreement with the value from the corresponding TEM images.Moreover,the vertical distances between the red arrows and between the green arrows respectively characterize the altitudes of the protuberant part and the sunk part of the twists with respect to the substrate. Referring to the complementary TEM dimension measurements, the former(17.9(0.5nm)corresponds to the width of the twisting ribbon,and the latter(11.2(0.5nm)corresponds to the thickness of the ribbon.From XRD,the height value of the C12-A (11-17)bilayer is about4.0nm(Figure S1in the Supporting Information),which might be a little smaller than the value in aqueous solution because of the drying states. Therefore,the ribbon with a thickness of∼11nm might comprise two bilayer units and a gapfilled with water molecules and counterions between them.Similar structure has been reported for chiral gemini surfactant16-2-16.34Such a kind of bilayer structure has not been found in the C12-A (11-17) sample at pH3.0by XRD.The variation infibril morphology at different solvent conditions should arise from the different manners of the intermolecular interactions within the self-assemblies,as il-lustrated in Figure6.At pH3.0,the hydrogen bond could form only between the residues next to the alkyl tails,and the outer part of the peptide moiety adopts the secondary structure of a flexible random coil.The two positive imidazolyl groups in the middle of the peptide increase the electrostatic repulsion between the neighboring head groups.Hence,the peptide-amphiphile molecules may organize into a cylinder with relatively high curvature.Moreover,the micelle surface is covered by neutral residues,which could lead to further aggregation of the cylindrical micelles into nanotapes through lateral association. Differently,at pH10.0the two negative carboxyl groups separate apart and the one at the terminal of the molecule is ratherflexible,which weakens the intermolecular repulsion.The peptide moiety adopts a -sheet conformation and the high extent of hydrogen bonding could drag the head groups closer. Thus,the curvature of the self-assembly is greatly reduced to a flat bilayer structure.Previous studies reported that amyloid polymorphism could arise from the variation in hydration states of the peptide,36the molecular conformation and packing within each protofilament,37-39and lateral association manners between protofilament units,40,41where many questions still remain unanswered.42Here,the results provide additional proof of the fibril polymorphism,and the proposed mechanism underlying the morphological variation of C12-A (11-17)self-assemblies with pH may be helpful for understanding the molecule-level structural basis of amyloidfibrilpolymorphism.Figure6.Schematic illustration of the intermolecular interaction within the C12-A (11-17)self-assemblies at pH 3.0(top)and pH10.0 (bottom).Yellow dashes represent hydrogen bonds.Self-assembly of Peptide-Amphiphile C12-A (11-17)J.Phys.Chem.B,Vol.113,No.25,20098543ConclusionsThe self-assembly of peptide-amphiphile C12-A (11-17) in aqueous solution has been studied in comparison with amyloid -peptide fragment A (11-17).A (11-17)is generally a hydrophilic peptide sequence and is not able to assemble into characteristic amyloidfibrils as other A fragments.By introduction of a long alkyl chain to the N-terminal of this sequence,the created peptide-amphiphile C12-A (11-17) displays the prominent biological features of A (1-40)in the morphology of the self-assemblies,such as rodlikefibrils,fibril bundles,and twisted ribbons,varying with pH condition.It implies the great importance of the hydrophobic interaction exerted upon amyloidfibrillogenesis and the significance of solvent condition on the amyloid polymorphism.Additionally, this work may help to guide the design of biomaterial scaffold-ings by inscribing biological signals in the self-assemblies. Acknowledgment.We are grateful forfinancial support from the National Natural Science Foundation of China and National BasicResearchProgramofChina(Grants20633010,2005cb221300). We gratefully acknowledge the Institute of Biophysics of the Chinese Academy of Sciences for access to the transmission electron microscope(FEI Tecnai20).Supporting Information Available:XRD spectra at pH3.0 and10.0,pH titration curve,and AFM image of the twisted fibrils at pH10.0in nine-color table form.This material is available free of charge via the Internet at . References and Notes(1)Whitesides,G.M.;Boncheva,M.Proc.Natl.Acad.Sci.U.S.A. 2002,99,4769.(2)Zhang,S.;Marini,D.M.;Hwang,W.;Santoso,S.Curr.Opin. Chem.Biol.2002,6,865.(3)Aggeli,A.;Bell,M.;Boden,N.;Keen,J.N.;McLeish,T.C.B.; Nyrkova,I.;Radford,S.E.;Semenov,A.J.Mater.Chem.1997,7,1135.(4)Silva,h.A.;Czeisler,C.;Niece,K.L.;Beniash,E.;Harrington,D.A.;Kessler,J.A.;Stupp,S.I.Science2004,303,1352.(5)Hartgerink,J.D.;Beniash,E.;Stupp,S.I.Science2001,294,1684.(6)Holmes,T.C.;Lacalle,S.d.;Su,X.;Liu,G.;Rich,A.;Zhang,S. Proc.Natl.Acad.Sci.U.S.A.2000,97,6728.(7)Zhang,S.;Holmest,T.;Lockshin,C.;Rich,A.Proc.Natl.Acad. Sci.U.S.A.1993,90,3334.(8)Lu,K.;Jacob,J.;Thiyagarajan,P.;Conticello,V.P.;Lynn,D.G. J.Am.Chem.Soc.2003,125,6391.(9)Hong,Y.;Legge,R.L.;Zhang,S.;Chen,P.Biomacromolecules 2003,4,1433.(10)Zhang,S.Biotechnol.Ad V.2002,20,321.(11)Paramonov,S.E.;Jun,H.-W.;Hartgerink,J.D.J.Am.Chem.Soc. 2006,128,7291.(12)Yamada,N.;Komatsu,T.;Yoshinaga,H.;Yoshizawa,K.;Edo, S.;Kunitake,M.Angew.Chem.,Int.Ed.2003,42,5496.(13)Ganesh,S.;Prakash,S.;Jayakumar,R.Biopolymers2003,70,346.(14)Yamada,N.;Ariga,K.;Naito,M.;Matsubara,K.;Koyama,E. J.Am.Chem.Soc.1998,120,12192.(15)Adams,D.J.;Holtzmann,K.;Schneider,C.;Butler,ngmuir 2007,23,12729.(16)Maltzahn,G.v.;Vauthey,S.;Santoso,S.;Zhang,ngmuir2003, 19,4332.(17)Vauthey,S.;Santoso,S.;Gong,H.;Watson,N.;Zhang,S.Proc. Natl.Acad.Sci.U.S.A.2002,99,5355.(18)Marini,D.M.;Hwang,W.;Lauffenburger,D.A.;Zhang,S.;Kamm, R.D.Nano Lett.2002,2,295.(19)Dong,H.;Paramonov,S.E.;Aulisa,L.;Bakota,E.L.;Hartgerink, J.D.J.Am.Chem.Soc.2007,129,12468.(20)Dexter,A.F.;Middelberg,A.P.J.J.Phys.Chem.C2007,111, 10484.(21)Nilsson,G.;Gustafsson,M.;Vandenbussche,G.;Veldhuizen,E.; Griffiths,W.J.;Sjo¨vall,J.;Haagsman,H.P.;Ruysschaert,J.-M.;Robertson,B.;Curstedt,T.;Johansson,J.Eur.J.Biochem.1998,255,116.(22)Hartgerink,J.D.;Beniash,E.;Stupp,S.I.Proc.Natl.Acad.Sci. U.S.A.2002,99,5133.(23)Stendahl,J.C.;Rao,M.S.;Guler,M.O.;Stupp,S.I.Ad V.Funct. Mater.2006,16,499.(24)Dexter,A.F.;Middelberg,A.P.J.Ind.Eng.Chem.Res.2008,47, 6391.(25)Clippingdale,A.B.;Wade,H.D.;Barrow,C.J.J.Peptide Sci. 2001,7,227.(26)Janek,K.;Rothemund,S.;Gast,K.;Beyermann,M.;Zipper,J.; Fabian,H.;Bienert,M.;Krause,E.Biochemistry2001,40,5457.(27)Marcinowski,K.J.;Shao,H.;Clancy,E.L.;Zagorski,M.G.J.Am. Chem.Soc.1998,120,11082.(28)Wood,S.J.;Wetzel,R.;Martin,J.D.;Hurle,M.R.Biochemistry 1995,34,724.(29)Danielsson,J.;Jarvet,J.;Damberg,P.;Gra¨slund,A.Biochemistry 2004,43,6261.(30)Balbach,J.J.;Ishii,Y.;Antzutkin,O.N.;Leapman,R.D.;Rizzo, N.W.;Dyda,F.;Reed,J.;Tycko,R.Biochemistry2000,39,13748.(31)Wang,J.;Zhu,S.;Xu,C.Biochemistry,Third Edition;Advanced Education Press:Beijing,2002.(32)Cao,M.;Han,Y.;Wang,J.;Wang,Y.J.Phys.Chem.B2007, 111,13436.(33)Behanna,H.A.;Donners,J.J.J.M.;Gordon,A.C.;Stupp,S.I. J.Am.Chem.Soc.2005,127,1193.(34)Oda,R.;Artzner,F.;Laguerre,M.;Huc,I.J.Am.Chem.Soc.2008, 130,14705.(35)Baumann,M.K.;Textor,M.;Reimhult,ngmuir2008,24, 7645.(36)Dzwolak,W.;Grudzielanek,S.;Smirnovas,V.;Ravindra,R.; Nicolini,C.;Jansen,R.;Loksztejn,A.;Porowski,S.;Winter,R.Biochemistry 2005,44,8948.(37)Petkova,A.T.;Leapman,R.D.;Guo,Z.;Yau,W.-M.;Mattson, M.P.;Tycko,R.Science2005,307,262.(38)Madine,J.;Jack,E.;Stockley,P.G.;Radford,S.E.;Serpell,L.C.; Middleton,D.A.J.Am.Chem.Soc.2008,130,14990.(39)Radovan,D.;Smirnovas,V.;Winter,R.Biochemistry2008,47, 6352.(40)Goldsbury,C.;Frey,P.;Olivieri,V.;Aebi,U.;Mu¨ller,S.A.J. Mol.Biol.2005,352,282.(41)Uversky,V.N.;Kabanov,A.V.;Lyubchenko,Y.L.J.Proteome Res.2006,5,2505.(42)Kodali,R.;Wetzel,R.Curr.Opin.Struct.Biol.2007,17,48. JP904289Y8544J.Phys.Chem.B,Vol.113,No.25,2009Deng et al.。
蛋白质及多肽分子自组装

蛋白质及多肽分子自组装北京大学力学系生物医学工程专业2003级,郭瑾摘要:自组装已成为合成一系列新型纳米材料的一种有效且有发展前景的方法。
本文将就蛋白质/多肽分子的组装方式:多肽纳米管、多肽纤维、蛋白质网络等做出具体介绍。
关键词:自组装 蛋白质 多肽 多肽纳米管 多肽纤维 蛋白质网络自组装已成为合成一系列新型纳米材料的一种有效且有发展前景的方法。
在自组装的过程中,原子、分子、粒子和其他基本单元在系统能量的驱动下具有组装形成功能性结构的能力。
自组装也指如果体系拆分成相应的亚单元,在适当的条件下,这些亚单元会混合重新形成完整结构。
对自组装过程,最重要的驱动力是亚单元间的相互作用能,无论这些亚单元是原子、分子或粒子。
分子自组装是在自由扩散的情况下,分子通过非共价键特异性结合形成有序的高级结构。
很多分子都可以自组装【1】:有机分子,蛋白质,多肽,DNA。
1981年Eric Drexler提出“bottom-up”式的构建纳米期间的方法,他指出以蛋白质作为构建单元通过自组装形成高级结构。
蛋白质或多肽分子自组装主要依靠氢键、静电相互作用、疏水相互作用等次级键的作用。
它们组装的形态主要有以下几种:1.多肽纳米管多肽纳米管目前采用以下几种可能的方式【2】:(1)折叠片层卷曲成中空的螺旋状结构(螺旋状卷曲式结构,图1a)(2)棍棒结构组装成筒状的分子束结构(筒状束式结构,图1b)(3)大环分子堆砌成连续管状结构(环状管式结构,图1c)(4)扇形或契形分子形成碟片状结构堆砌成碟片状柱式结构(图1d)近年来,有些学者又提出了一些新的纳米管模型。
Meital Reches等【3】在研究非常短的芳香肽形成淀粉样纤维的稳定性时观察到了纳米管的形成。
在理论和实验的基础上,他们认为芳香残基的堆积在分子识别及自组装成纤维的过程中起重要作用。
由于芳香部分有受限的几何形状,而且之间还有吸引力,因此该部分可以提供形成有序结构而需要的有序性,方向性。
超分子化学读物

超分子化学读物以下是一些推荐的超分子化学读物:1.《超分子化学》(Supramolecular Chemistry)作者:Jonathan W. Steed和Jerry L. Atwood这本书是超分子化学领域的经典教材,详细讲解了超分子化学的基本原理、方法和应用。
涵盖了分子识别、自组装、超分子组装、功能材料等内容。
2.《超分子化学导论》(Supramolecular Chemistry: An Introduction)作者:David J. Amabilino这本书提供了对超分子化学领域的综合介绍。
书中包括对非共价相互作用的讨论,涵盖了超分子结构、自组装、分子识别、超分子材料等主题。
3.《分子自组装的物理原理》(Physical Principles of the Self-assembly Process)作者:Tchavdar N. Todorov,Michael J. Smith和 Alberto Striolo该书从物理学和化学的角度探讨了分子自组装的原理和机制。
内容包括自组装的理论模型、表面活性剂和胶束、薄膜自组装等。
4.《功能自组装体化学》(Functional Self-Assembling Peptides: Chemistry and Materials Science)作者:Izumi Hirota这本书重点关注自组装肽的化学和材料科学应用。
内容包括自组装肽的合成、性质和应用,以及与其他纳米材料和生物分子的相互作用。
5.《超分子化学的最新进展》(Recent Advances in Supramolecular Chemistry)作者:Pavel Anzenbacher和Nicholas J. Turro这本书收集了一系列关于超分子化学的最新研究进展,涵盖了分子设计与合成、功能材料和生物应用等领域的前沿研究成果。
希望以上推荐的读物对您有所帮助!。
SELF-ASSEMBLING PEPTIDES INCORPORATING MODIFICATIO
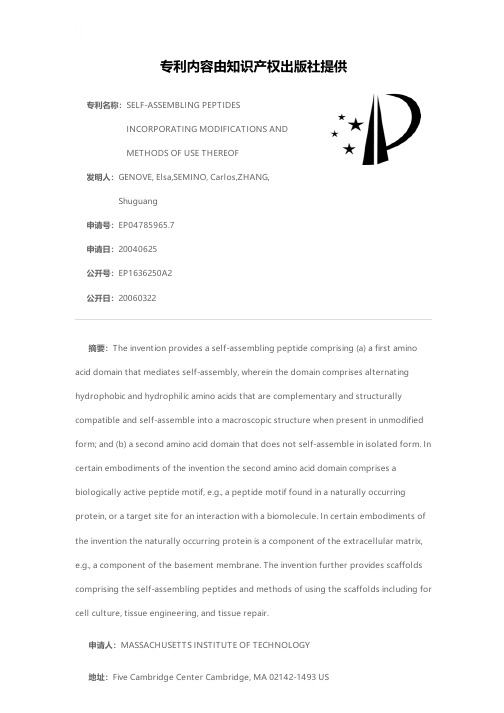
专利名称:SELF-ASSEMBLING PEPTIDESINCORPORATING MODIFICATIONS ANDMETHODS OF USE THEREOF发明人:GENOVE, Elsa,SEMINO, Carlos,ZHANG, Shuguang申请号:EP04785965.7申请日:20040625公开号:EP1636250A2公开日:20060322专利内容由知识产权出版社提供摘要:The invention provides a self-assembling peptide comprising (a) a first amino acid domain that mediates self-assembly, wherein the domain comprises alternating hydrophobic and hydrophilic amino acids that are complementary and structurally compatible and self-assemble into a macroscopic structure when present in unmodified form; and (b) a second amino acid domain that does not self-assemble in isolated form. In certain embodiments of the invention the second amino acid domain comprises a biologically active peptide motif, e.g., a peptide motif found in a naturally occurring protein, or a target site for an interaction with a biomolecule. In certain embodiments of the invention the naturally occurring protein is a component of the extracellular matrix, e.g., a component of the basement membrane. The invention further provides scaffolds comprising the self-assembling peptides and methods of using the scaffolds including for cell culture, tissue engineering, and tissue repair.申请人:MASSACHUSETTS INSTITUTE OF TECHNOLOGY地址:Five Cambridge Center Cambridge, MA 02142-1493 US国籍:US代理机构:Gambell, Derek 更多信息请下载全文后查看。
多肽分子自组装
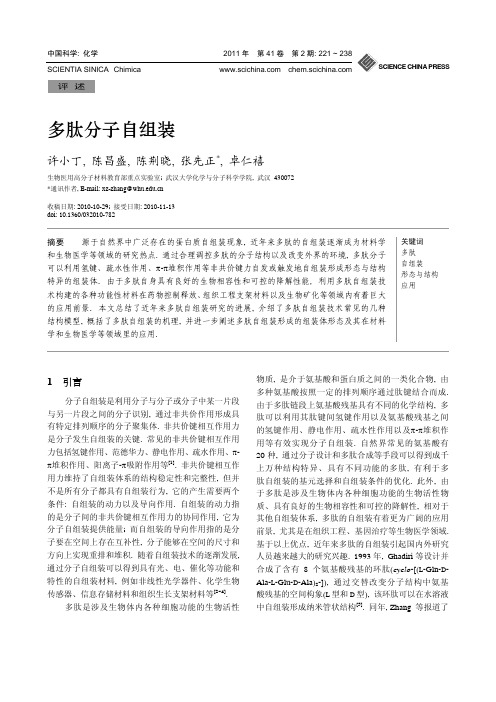
中国科学: 化学 2011年第41卷第2期: 221 ~ 238 SCIENTIA SINICA Chimica 《中国科学》杂志社SCIENCE CHINA PRESS评述多肽分子自组装许小丁, 陈昌盛, 陈荆晓, 张先正*, 卓仁禧生物医用高分子材料教育部重点实验室; 武汉大学化学与分子科学学院, 武汉 430072 *通讯作者, E-mail: xz-zhang@收稿日期: 2010-10-29; 接受日期: 2010-11-13doi: 10.1360/032010-782摘要源于自然界中广泛存在的蛋白质自组装现象, 近年来多肽的自组装逐渐成为材料学和生物医学等领域的研究热点. 通过合理调控多肽的分子结构以及改变外界的环境, 多肽分子可以利用氢键、疏水性作用、π-π堆积作用等非共价键力自发或触发地自组装形成形态与结构特异的组装体. 由于多肽自身具有良好的生物相容性和可控的降解性能, 利用多肽自组装技术构建的各种功能性材料在药物控制释放、组织工程支架材料以及生物矿化等领域内有着巨大的应用前景. 本文总结了近年来多肽自组装研究的进展, 介绍了多肽自组装技术常见的几种结构模型, 概括了多肽自组装的机理, 并进一步阐述多肽自组装形成的组装体形态及其在材料学和生物医学等领域里的应用. 关键词多肽自组装形态与结构应用1 引言分子自组装是利用分子与分子或分子中某一片段与另一片段之间的分子识别, 通过非共价作用形成具有特定排列顺序的分子聚集体. 非共价键相互作用力是分子发生自组装的关键. 常见的非共价键相互作用力包括氢键作用、范德华力、静电作用、疏水作用、π- π堆积作用、阳离子-π吸附作用等[1]. 非共价键相互作用力维持了自组装体系的结构稳定性和完整性, 但并不是所有分子都具有自组装行为, 它的产生需要两个条件: 自组装的动力以及导向作用. 自组装的动力指的是分子间的非共价键相互作用力的协同作用, 它为分子自组装提供能量; 而自组装的导向作用指的是分子要在空间上存在互补性, 分子能够在空间的尺寸和方向上实现重排和堆积. 随着自组装技术的逐渐发展, 通过分子自组装可以得到具有光、电、催化等功能和特性的自组装材料, 例如非线性光学器件、化学生物传感器、信息存储材料和组织生长支架材料等[2~4].多肽是涉及生物体内各种细胞功能的生物活性物质, 是介于氨基酸和蛋白质之间的一类化合物, 由多种氨基酸按照一定的排列顺序通过肽键结合而成. 由于多肽链段上氨基酸残基具有不同的化学结构, 多肽可以利用其肽键间氢键作用以及氨基酸残基之间的氢键作用、静电作用、疏水性作用以及π-π堆积作用等有效实现分子自组装. 自然界常见的氨基酸有20种, 通过分子设计和多肽合成等手段可以得到成千上万种结构特异、具有不同功能的多肽, 有利于多肽自组装的基元选择和自组装条件的优化. 此外, 由于多肽是涉及生物体内各种细胞功能的生物活性物质、具有良好的生物相容性和可控的降解性, 相对于其他自组装体系, 多肽的自组装有着更为广阔的应用前景, 尤其是在组织工程、基因治疗等生物医学领域. 基于以上优点, 近年来多肽的自组装引起国内外研究人员越来越大的研究兴趣. 1993年, Ghadiri等设计并合成了含有8个氨基酸残基的环肽(cyclo-[(L-Gln-D- Ala-L-Glu-D-Ala)2-]), 通过交替改变分子结构中氨基酸残基的空间构象(L型和D型), 该环肽可以在水溶液中自组装形成纳米管状结构[5]. 同年, Zhang 等报道了许小丁等: 多肽分子自组装222一种可以自组装形成水凝胶膜的离子互补型十六肽[6]. 随后, 一系列具有自组装行为的多肽相继被报道[7~21]. 多肽自组装可以分为自发型自组装和触发型自组装. 自发型自组装是指多肽溶解在水溶液中后, 可以自发地形成组装体. 如Zhang 等报道的由精氨酸 (R)残基、天冬氨酸(D)残基和丙氨酸(A)残基交替排列的离子互补型RAD16系列肽[6~8, 22]以及可以自组装形成纳米管、囊泡等结构的脂质体型小分子多肽[23, 24]. 触发型多肽自组装是指通过改变外界环境如温度、pH 、离子浓度等引导的自组装. 目前大部分的多肽自组装研究集中在触发型自组装, 因为这类自组装具有可逆性, 为多肽自组装技术的潜在应用提供了良好的可控性. 目前已报道的触发型多肽自组装主要包括温度敏感[25, 26]、pH 敏感[27, 28]、光敏感[29, 30]以及配体-受体敏感[31, 32]等类型的自组装. 事实上, 无论是自发型还是触发型, 其自组装都是基于二级结构如α-螺旋(α-helix)、β-折叠(β-sheet )的形成或者其自身分子结构的两亲性. 本文从多肽二级结构的转变以及其自身结构的两亲性逐一阐述多肽自组装的机理、自组装体的形貌及其应用.2 基于二级结构形成的多肽自组装多肽的一级结构即为自身的化学结构, 当把多肽溶解在水溶液中, 多肽分子可以自发或触发地向二级结构转变. 这种空间构象的转变往往导致多肽自组装行为的发生. 多肽自组装过程中常见的二级结构主要包括α-螺旋(α-helix)、β-折叠(β-sheet )、β-发夹(β-hairpin )等.2.1 α-螺旋(α-Helix )α-螺旋是多肽类分子主要的二级结构, 空间上表现为多肽链段上肽键通过氢键作用形成的单一的螺旋结构(图1(a)). 在构建α-螺旋结构时, 由于每一个螺旋状的旋转需要大约3.6个氨基酸残基, 因此多肽链段上3到4个氨基酸残基组成的多肽片段需要具有类似的化学性质, 如亲疏水性等. 由于α-螺旋的热动力学不稳定性, 在溶液中往往难以稳定的螺旋形式图1 多肽α-螺旋空间结构(a)以及Acetyl-EA C ARVAibAA C EAAARQ-NH 2肽[33](b)、FK-4-X 和FK-11-X 肽[37](c)光敏感自组装行为示意图中国科学: 化学 2011年 第41卷 第2期223存在. 因此, 稳定α-螺旋构象对于此类多肽的自组装研究具有极其重要的意义. 常用的方法有化学交联在α-螺旋结构中同一侧面的氨基酸残基、氢键配对、金属配位以及盐桥作用等[28, 33~41]. 例如, Kumita 等[33]利用多肽固相合成技术得到了含有两个半胱氨酸(C)残基的十六肽(acetyl-EA C ARVAibAA C EAAARQ- NH 2, 两个半胱氨酸残基分别位于i 和i +7位). 如图1(b)所示, 用含有偶氮苯基团的交联剂对两个半胱氨酸残基进行交联后, 由于在光照的条件下偶氮苯基团可以实现反式到顺式的转变, 导致该肽在水溶液中的α-螺旋结构大大增加并趋于稳定, 这样也使得其自组装行为具有光敏感特性. 同时, 他们还进一步研究了两个半胱氨酸残基分别位于i 和i +4位(acetyl- EAAAREA C ARE C EAARQ-NH 2, FK-4-X)以及i 和i + 11位(acetyl-EA C AREAAAREAA C RQ-NH 2, FK-11-X) 的两种肽的自组装行为[34]. 通过含有偶氮苯基团的交联剂对两个半胱氨酸残基进行交联, 顺式的偶氮苯基团有利于FK-4-X 肽在水溶液中形成稳定的α- 螺旋结构, 而反式的偶氮苯基团则有利于FK-11-X 肽在水溶液中形成稳定的α-螺旋结构(图1(c)). Mihara 等[42]设计合成了Ac-AAEALLKAHAELLAKA- AGGGC-NH 2二十一肽. 在水溶液中, 多肽链段上谷氨酸(E)残基和赖氨酸(K)残基间的盐桥作用在一定程度上能够稳定α-螺旋结构. 同时该种多肽结构上半胱氨酸残基可以通过二硫键交联形成二聚的H2α-17肽. 这种新形成的H2α-17肽结构中的两个组氨酸(H)残基通过与血红素识别形成金属配位桥联的平行α-螺旋链, 导致α-螺旋的稳定性显著增强, 能够自组装形成稳定的纳米聚集体. 另外Lee 等[43]巧妙地利用多肽分子的β-折叠(β-sheet )结构来增强和稳定α-螺旋构像. 研究发现, 孤立的AAAAKAAAAK 多肽片段在水溶液中只有部分形成α-螺旋结构, 而将AAAAKAAAAK 多肽片段与WKWEWKWEW 多肽片段相连形成环状多肽后, WKWEWKWEW 多肽片段形成的β-折叠有序排列能够有效地诱导AAAAK- AAAAK 多肽片段形成稳定的α-螺旋结构, 从而使得该环肽在水溶液中组装成纳米棒、球状纳米粒子等结构.除了α-螺旋, 近年来研究发现, 部分多肽的自组装是基于多股α-螺旋即卷曲螺旋(coiled-coil)的超二级结构[15, 16, 44~46]. 其空间上表现为两股或多股α-螺旋结构之间通过氢键作用、静电作用以及疏水性作用形成的超螺旋结构. 用于构建卷曲螺旋结构的多肽分子结构中正常含有3到4个由7个氨基酸残基组成的基本重复单元(图2(a)中的a~g). 其中, a, d, e, g 四图2 (a)多肽卷曲螺旋超二级结构; (b)含有SGDLENEVAQLEREVRSLEDEAAELEQKVSRLKNEIEDLKAE 序列的七十二肽形成的卷曲螺旋结构[47]; (c)该七十二肽在pH 为4.5, 7.4以及11.2水溶液的圆二色谱图(208, 222 nm 为α-螺旋特征峰)[50]; (d)该七十二肽与金纳米粒子键合后的透射电镜图[51]许小丁等: 多肽分子自组装224个位置上的氨基酸残基之间的相互作用是构建卷曲螺旋模型的内在作用力; a, d 两个位置上氨基酸残基之间的作用力为疏水性作用, 而e, g 两个位置上氨基酸残基则可以通过静电作用来达到调节卷曲螺旋结构内核的疏水性强弱. 因此, 如果改变外界的pH 值, e, g 两个位置的氨基酸残基可以进行质子化, 它们之间的静电排斥作用使得卷曲螺旋结构趋于不稳定. 事实上, 很多研究表明基于卷曲螺旋结构的多肽自组装都具有pH 敏感性[47~50]. 例如, Stevens 等[50]合成了一种含有半胱氨酸端基的七十六肽, 其中42个氨基酸序列(SGDL E N E VAQL E R E VRSL E D E AAEL E Q K VSRL K N E IEDL K A E )可以形成卷曲螺旋结构. 如图2(b)所示, 由于d 位置是亮氨酸(L)残基而e, g 两位置为谷氨酸(E)残基, 在酸性水溶液中(pH 4.5), 该肽可以形成卷曲螺结构, 而且e, g 两位置上谷氨酸残基间氢键作用可以稳定卷曲螺旋结构, 使得该肽可以自组装形成纳米纤维. 使用圆二色谱仪跟踪监测时发现(图2(c)), 升高溶液的pH 值至中性(pH 7.4)或碱性(pH 11.2)后, e, g 两位置上谷氨酸残基质子化, 它们之间的静电排斥作用使得卷曲螺旋结构趋于不稳定, 会破坏自组装形成的纳米纤维结构. 基于上述的pH 敏感性, 他们更进一步利用半光氨酸端基上的巯基与金纳米粒子共价键结合[51]. 在中性条件下(pH 7.4), 由于卷曲螺旋结构不稳定, 金纳米粒子均匀分散在水溶液中; 而在酸性条件下(pH 4.5), 多肽的自组装可以使金纳米粒子迅速在纤维表面聚集, 达到还原金的目的(图2(d)).与Stevens 等不同, Woolfson 等在保持d 位置为亮氨酸(L)残基的基础上, 在e, g 两位置上引入谷氨酸(E)以及赖氨酸(K)残基, 得到一系列离子互补型二十八肽(图3(a))[52]. 在中性水溶液中, 这一系列肽可以利用e, g 两位置上谷氨酸以及赖氨酸残基之间静电吸引作用构建稳定的卷曲螺旋结构, 并自组装形成纳米纤维. 升高溶液的离子浓度后, 谷氨酸以及赖图3 (a)卷曲螺旋结构中e, g 两位置上位谷氨酸(E)以及赖氨酸(K)残基的几种多肽[52]; (b)SAF-p1和SAF-p2a 两种肽共同自组装形成的微米级纤维透射电镜图[53]; (c)SAF-p1和SAF-p2a 两种肽在自组装过程中初始形成的原纤维表面异性电荷的静电吸引作用示意图[54]中国科学: 化学 2011年 第41卷 第2期225氨酸残基上的电荷被屏蔽, 卷曲螺旋结构转变为无规卷曲, 进而破坏了纳米纤维自组装结构. 在此基础上, 他们还进一步研究发现, 卷曲螺旋结构中b 位置分别为天冬氨酸和精氨酸的两种肽(图3(a)中SAF-p1和SAF-p2a)可以在水溶液中共同自组装形成稳定的微米级纤维(图3(b))[53, 54]. 通过高倍透射电镜观测可以发现这些微米级纤维是由很多个纳米纤维定向聚集而成. 其聚集的驱动力是SAF-p1和SAF-p2a 两种肽在自组装过程中初始形成的原纤维表面异性电荷的静电吸引作用(图3(c)).2.2 β-折叠(β-Sheet )β-折叠也是多肽类分子常见的二级结构, 空间结构表现为多肽链段通过平行(称为平行β-折叠)或反平行(称为反平行β-折叠)方式排列形成的薄片, 其内部作用力主要为多肽链段上肽键间的氢键作用(图4(a)). 在众多的多肽自组装研究中, 反平行β-折叠结构居多. 这是因为反平行β-折叠结构中多肽链段之间氢键距离(图4(b))相对平行β-折叠中氢键距离较短(图4(c)), 氢键作用相对较强. 研究表明, 许多疾病, 如Alzheimer 和Parkinson 等与体内蛋白β-折叠空间结构所导致的沉淀聚集有关[55~57]. 基于β-折叠的多肽自组装研究很多, 其中以亲疏水氨基酸残基相互穿插构成的多肽居多, 一个典型代表为Lego 肽[6~8, 58~63]. 这种肽由极性和非极性氨基酸残基交替排列组成, 类似Lego 玩具上凸出的栓和凹陷的孔洞而得名. 由于Lego 肽这种规则的分子结构(极性和非极性氨基酸交替排列), 多肽链段易在空间上通过氢键作用相互排列堆积形成β-折叠的二级结构. Yokoi 等[22]设计了由带正电荷的精氨酸(R)残基、带负电荷的天冬氨酸(D)残基和不带电荷的丙氨酸(A )残基交替排列的RADA16-I 肽([COCH 3]-RADARADARADARA-DA- [CONH 2], 图4(d)). 如图4(e)所示, 在水溶液中, 疏水的丙氨酸残基迅速彼此靠拢聚集以降低体系的能量, 而能够电离的天冬氨酸残基和精氨酸残基则通过静电作用相互吸引排列在组装体的外层. 由于丙氨酸残基是靠疏水作用力相结合, 而非化学键键合, 丙氨酸残基可以横向滑移以减少其与水分子的接触,图4 (a)多肽β-折叠二级结构; (b)多肽β-折叠二级结构中平行氢键作用; (c)多肽β-折叠二级结构中反平行氢键作用; (d)Lego 型RADA16-I 肽分子3D 模拟结构[22]; (e)Lego 型RADA16-I 肽自组装过程中丙氨酸残基横向滑移形成规整的具有β-折叠结构的纳米纤维[22]许小丁等: 多肽分子自组装226最终使肽链的疏水面完全契合形成规整的具有β-折叠结构并自组装形成纳米纤维. 目前, RADA16-I 肽已经商品化(PuraMatrix), 应用于多种组织和细胞培养[8, 62, 63].除了上述RADA16-I 肽, Lego 肽还有大量其他的 分子结构. 例如, Rapaport 等[64]报道的Pro-Glu-(Phe- Glu)n -Pro (n = 4, 5或7)肽, 由带负电荷的亲水谷氨酸残基Glu 和疏水的苯丙氨酸残基Phe 构成, 在空气和水的界面能以β-折叠的结构自组装形成单分子层. 类似的, Kamm 等[65~67]合成的八肽FKFEFKFE(KFE8)中, 赖氨酸(K)残基带正电荷, 谷氨酸(E)残基带负电荷, 而苯丙氨酸(F)残基不带电荷, 组成了典型的亲疏水交替肽. 通过实验观测与模拟计算发现, KFE8肽能够以双螺旋状β-折叠结构自组装形成较规则的纳米纤维. 在pH 值为4时, 纳米纤维可以在水溶液中迅速相互缠绕形成KFE8肽的超分子凝胶. Epand 等[68]报道的Ac-(LeuLysLysLeu)5-NHEt 和Ac-(LysLeu)10- NHEt 肽也能够在水溶液中以β-折叠结构自组装形成纳米纤维.除了自组装形成纳米纤维外, 研究发现多肽分子还可以利用其β-折叠的二级结构自组装形成纳米管, 囊泡等组装体. 例如, Ghadiri 等[5]最早设计并合成的含有8个氨基酸残基的环肽(cyclo -[(L -Gln-D - Ala-L -Glu-D -Ala)2-]), 如图5(a)所示, 通过交替改变分子结构中丙氨酸残基的空间构象(L 型和D 型). 该环肽可以在水溶液中自组装形成具有β-折叠结构的纳米管. Couet 等[69]在环肽cyclo -(L -Lys-D -Ala-L -Asp- D -Ala-L -Lys-D -Ala-L -Lys-D -Ala)结构上引入异丁基溴功基. 在水溶液中, 该种环肽可以自组装形成具有β-折叠结构的纳米管. 如图5(b)所示, 由于异丁基溴功基分布在纳米管的表面, 该种纳米管可以作为模板进行原子转移自由基聚合(ATRP), 形成一种多肽-聚合物复合纳米材料. Reiriz 等[70]利用固相合成技术将C 60接到含有八个氨基酸残基的环肽上. 如图5(c)所图5 (a)Ghadiri 等最早设计并合成的环肽(cyclo -[(L -Gln-D -Ala-L -Glu-D -Ala)2-]自组装形成具有β-折叠结构的纳米管[5]; (b)含有异丁基溴功能基的cyclo -(L -Lys-D -Ala-L -Asp-D -Ala-L -Lys-L -Ala-L -Lys-D -Ala)环肽自组装形成具有β-折叠结构的纳米管并引发N -异丙基丙烯酰胺聚合形成多肽-聚合物复合纳米材料[69]; (c)共价键结合C 60的环肽在水溶液中自组装形成含有单层C 60的纳米管[70]中国科学: 化学 2011年 第41卷 第2期227示, 在水溶液中该肽能够利用其β-折叠结构自组装形成含有单层C60的纳米管. 近年来, 由环肽利用其β-折叠结构自组装形成的纳米管在生物传感器[71]、光器件[72]以及电化学器件[73]等方面都有着广泛的应用.研究表明, 不仅单一的多肽链段能够以β-折叠结构进行自组装, 两种或多种多肽也可通过分子间的非共价键相互作用共同自组装形成具有β-折叠结构的组装体. Ramachandran 等[74, 75]分别研究了阳离子型acetyl-KWKVKVKVKVKVKVK-NH 2-(KVW15)、acetyl-WKVKVKVKVK-NH 2(KVW10)肽以及阴离子型acetyl-EWEVEVEVEV-NH 2(EVW10)肽在水溶液中的自组装行为. 把阳离子型和阴离子型肽简单地混合在水溶液中后, 分子间静电吸引作用可以使两种多肽分子结合在一起, 并共同自组装形成具有β-折叠结构的纳米纤维. 相对于单一阳离子型或阴离子型肽的自组装, 这种共同自组装并未改变溶液的pH 值和盐浓度, 因此在组织工程支架材料领域具有潜在的应用前景.2.3 β-发夹(β-Hairpin)β-发夹是多肽二级结构β-turn(β-转角)的衍变, 类似于β-折叠结构, 是由Schneider 等[76]提出的一种多肽结构模型. 空间结构表现为多肽链段上氨基酸残基通过氢键作用形成的U 形弯曲. 构建β-发夹结构要求多肽链段中必须含有能够发生弯曲的氨基酸序 列, 常见的是脯氨酸-甘氨酸(Pro-Gly)或者脯氨酸-苏氨酸(Pro-Thr)序列. Schneider 等利用多肽的固相合成技术先后制备了MAX1-7七种可以形成β-发夹结构的多肽(图6(a)). 在这些多肽分子结构中, 亲水性的赖氨酸(K)残基和疏水性的缬氨酸(V)氨基交替排列. 在酸性条件下, 质子化的赖氨酸残基之间的静电排斥作用使多肽分子不能形成β-发夹结构. 升高溶液的pH 值或增加溶液的离子浓度屏蔽静电排斥作用后, 多肽则可以形成以赖氨酸残基为内面、缬氨酸残基为外面的β-发夹结构, 并进一步利用其缬氨酸残基外面的疏水性作用自组装形成纳米纤维(图6(b))[76~78]. 由于MAX 系列肽可以在细胞培养液DMEM 中自组装形成多肽凝胶, 因而可以作为细胞生长支架材料[75]以及药物控释载体[80]. 同时, 由于MAX 系列肽中存在可电离的赖氨酸残基, 由MAX 系列肽构成的多肽凝胶还具有一定的抑菌功能[81]. Schneider 等还进一步对MAX 系列肽 进行化学修饰, 使得它们的自组装行为具有光[29]、温图6 (a)可以形成β-发夹空间结构的MAX1-7系列肽[76~78]; (b)MAX 系列肽通过构建β-发夹空间结构自组装形成纳米纤维和多肽凝胶[76]度[82]等敏感特性.3 基于两亲性分子结构的多肽自组装两亲性分子与水分子相互作用时倾向于将其亲水段裸露在外围与水分子形成交界面, 疏水段则向内聚集. 在多肽的自组装研究中, 研究人员将传统的两亲性概念引入到多肽自组装体系中. 常用的手段是将疏水性的基团引入到多肽链段的一端, 这样得到的多肽衍生物类似于表面活性剂或脂质体, 通常称之为两亲性多肽(peptide amphiphile). 相对于前面所述的利用多肽空间结构转变的自组装, 两亲性多肽需要的多肽链段相对较短, 例如在简单的甘氨酸-甘氨酸(GG)二肽一端引入9-芴甲氧羰基(FMOC)就可使其自组装形成纳米纤维[31]. 目前常用的疏水性基团主要包括脂肪族烷基链和芳香族功能基.3.1 以脂肪族烷基链为端基的两亲性多肽自组装 Kunitake 等最早提出了两亲性多肽的概念[83]. 如7(a)所示, 这种两亲性多肽包括一个疏水性的尾部(由一个或多个脂肪族烷基链组成)、连接区、间隔区许小丁等: 多肽分子自组装228以及亲水性的头部(由多肽链段组成). 连接区将疏水性尾部与亲水性头部相连, 而间隔区增加二者之间连接距离, 以避免头尾两部分在自组装过程中相互干扰. 这种两亲性多肽能在水溶液中进行亲水段向外、疏水段向内的有序排列. 通过合理调控其结构中四部分的成分以及长短可以得到胶束、囊泡、单层膜、纳米纤维、纳米管、纳米盘等组装体[12, 13, 84]. Gore 等[85]设计合成了类似于胶原蛋白三螺旋结构的两亲性多肽(图7(b)), 并研究疏水性烷基链长短、数目以及温度对该肽自组装行为的影响. 结果表明, 具有单个疏水性烷基链的两亲性多肽可以在水溶液中自组装形成球状胶束. 含有两个C12或C14疏水性烷基链的两亲性多肽同样可以自组装形成球状胶束(图7(c)); 增长两个疏水性烷基链长度至C16或C18时, 两亲性多肽可以自组装形成盘状胶束, 而且这些盘状胶束在室温下可以相互堆积形成绳状组装体(图7(d)). 此时, 升高体系的温度并冷却至室温后, 绳状组装体消失, 取而代之的是球状胶束.近年来, 许多研究人员对Kunitake 等提出的两亲性多肽概念进行简化并发现许多只有疏水性烷基链和亲水性的多肽链段组成的两亲性多肽也可以进 行自组装. 如图8(a)所示, 这类两亲性多肽可以通过烷基链的疏水性作用以及多肽链段的氢键作用(β-折叠二级结构)在水溶液进行自组装, 形成的组装体绝大部分为纳米纤维(由于这些纳米纤维具有规整的疏水性内核和亲水性外壳, 可称之为纤维状胶束[12]). Stupp 等对这一类型的两亲性多肽进行了系统、深入的研究, 包括在多肽链段中引入具有生物活性氨基酸序列, 例如具有细胞粘附特性的线性和环状精氨酸-甘氨酸-天冬氨酸(RGD)序列[13, 86~89], 以及能够促进神经突生长的异亮氨酸-赖氨酸-缬氨酸-丙氨酸-缬氨酸序列(IKVAV)[90~92]. 他们还针对这类两亲性肽的自组装形态, 采用计算模拟辅以实验观察的方式绘制出形态转变的“相图”[93, 94](图8(c)), 研究此类两亲性多肽分子在水溶液中的自组装形态变化与分子间作用力、溶液pH 值、溶液盐离子浓度等之间的关系, 较直观地阐述了两亲性多肽在不同条件下的自组装形态及结构模型. Paramonov 等[95]对肽链上N-甲基化进行修饰来调控氢键数目, 研究氢键作用对这类两亲性多肽自组装行为的影响. 研究发现, 减少肽链片段间的氢键作用将会使分子不易形成纳米纤维, 而更倾向于形成球形纳米胶束. 我们也考察了不同图7 (a)Kunitake 等两亲性多肽的结构模型[83]; (b)类似于胶原蛋白三螺旋结构的几种两亲性多肽分子结构[85]; (c), (d)该系列两亲性多肽自组装形成的组装体透射电镜图[85]中国科学: 化学 2011年 第41卷 第2期229图8 (a)由疏水性烷基链和亲水多肽链段组成的两亲性多肽自组装机理[12]; (b)计算模拟该类两亲性肽自组装结构 (εHH )相图, 其中各个区域分别代表自由分子(A)、球形胶束(B)、外围为β-折叠冠状结构的胶束(C)、圆柱状长纤维(D)、平行堆积β-折叠(E)、单层β-折叠(F)以及无规聚集体(G)[93, 94]的疏水性端基、氨基酸序列以及溶液的pH 对这一类型两亲性多肽自组装行为的影响[96, 97]. 研究发现, 在中性或碱性水溶液中, 由于两亲性多肽电离而存在静电排斥作用, 具有较短疏水性烷基链或较大体积疏水性端基(如FMOC 功能基)的两亲性多肽更加倾向于形成球状纳米胶束或囊泡, 其自组装行为与离子型表面活性剂类似. 对于具有较长疏水性烷基链的两亲性多肽, 由于其较强的疏水性作用有效抑制静电排斥作用, 两亲性多肽更加倾向于形成纳米纤维结构(图9(a)).含有疏水性烷基链的两亲性多肽除了可以自组装得到各种功能性多肽材料, 研究人员还在其多肽链段中引入带正电的氨基酸残基如精氨酸R 和赖氨酸K, 制备可以携带DNA 的两亲性多肽基因载体[98~100]. 相对于传统的基因载体, 如25 kDa 聚乙烯亚胺(PEI), 两亲性多肽基因载体可以有效降低载体毒性和提供靶向性. 我们[101]制备了一系列含有跨膜肽(8个连续的精氨酸序列)的两亲性多肽基因载体. 这些两亲性多肽能够与DNA 复合并共同自组装形成纳米组装体(图9(b)). 其基因转然效率随着疏水性烷基链的增长而显著增加. 其中含有RGD 靶向基团C 17H 35-CONH- GR 7RGD 肽负载DNA 的转染效率与25 kDa PEI 相当. Liu 等[102]将疏水性的烷基链改为胆固醇并在多肽链段引入TAT 肽(6个连续的精氨酸序列), 得到一种新型的两亲性多肽基因载体(图9(c)). 研究表明该两亲性多肽可以与DNA 紧密的复合, 并表达出较高的转染效率达到抗菌的效果. Tarwadi 等[103]采用带正电荷的赖氨酸K 、具有pH 缓冲作用的组氨酸H 以及可以通过氧化交联的半胱氨酸C 与一条烷基链构成两亲性肽. 研究表明, 其中的两种肽PalCK 2H 2和PalCK 3H 2(Pal: palmitoyl fatty chain C 16)可较好地复合DNA, 且转染效率较高, 能够有效地在细胞中进行基因传递. 而且通过调节组氨酸和赖氨酸的比例以及在多肽链段上的排列顺序, 还可进一步提高转染效率.除了上述含有疏水性尾部和亲水性头部的两亲。
- 1、下载文档前请自行甄别文档内容的完整性,平台不提供额外的编辑、内容补充、找答案等附加服务。
- 2、"仅部分预览"的文档,不可在线预览部分如存在完整性等问题,可反馈申请退款(可完整预览的文档不适用该条件!)。
- 3、如文档侵犯您的权益,请联系客服反馈,我们会尽快为您处理(人工客服工作时间:9:00-18:30)。
Abstract Biological self-assembly systems lie at the interface between molecular biology, chemistry, polymer science and engineering. The key elements in molecular self-assembly are chemical complementarity and structural compatibility. Several types of self-assembling peptides have been engineered. Type I peptides undergo intermolecular self-assembly, type II peptides undergo self-assembly and disassembly, i.e. intermolecular and intramolecular self-assembly under the influence of various conditions. Type III peptides undergo self-assembly on to surfaces. These self-assembling peptide systems are simple, versatile and easy to modify and to produce. These systems represent a significant advancement in the molecular engineering of protein fragments for diverse technological innovations. © 1999 Elsevier Science B.V. All rights reserved.
1381-5148 / 99 / $ – see front matter © 1999 Elsevier Science B.V. All rights reserved. PII: S1381-5148( 99 )00031-0
92
S. Zhang, M. Altman / Reactive & Functional Polymers 41 (1999) 91 – 102
*Corresponding author. Tel.: 1 1-617-258-7514, fax: 1 1-617258-0204. E-mail address: shuguang@ (S. Zhang)
Molecular self-assembly is the basis of polymers at the early stage before polymerization. Proteins and protein fragments have not been considered to be useful materials for traditional engineering science, although their potential has been recognized. Several recent discoveries and rapid developments in biotechnology, however, have rekindled the field of biological materials engineering [2–7]. In the last few years, considerable advances have been made in the use of peptides as building blocks to produce biological materials for a wide range of applications. A new class of oligopeptide based biological materials was serendipitously discovered from the self-assembly of ionic self-complementary oligopeptides [7–11]. A number of
Keywords: Cell attachment; Cell pattern formation; Ionic complementarity; Molecular switch; Neurite outgrowth; Peptide matrices; Structural transformation; Surface engineering
self-assembling peptides have been designed and analyzed (Table 1). These systematic analyses provided insight into the chemical and structural principles of peptide self-assembly. This class of biomaterials has considerable potential in a number of applications, including platforms or scaffolding for tissue engineering [9,12], drug delivery of protein and peptide medicine, as well as biological surface engineering [13]. These peptides are short, simple to design, extremely versatile and easy to synthesize. Three types of self-assembling peptides have been systematically studied thus far. It is believed additional different types will be discovered and developed over time. Type I peptides form beta-sheet structures in aqueous solution because they contain two distinct surfaces, one hydrophilic, the other hydrophobic. The unique structural feature of these peptides is that they form complementary ionic bonds with regular repeats on the hydrophilic surface. The complementary ionic sides have been classified into several moduli, i.e. modulus I, II, III, IV, etc., and mixed moduli. This classification is based on the hydrophilic surface of the molecules that have alternating 1 and 2 charged amino acid residues, either alternating by 1, 2, 3, 4 and so on. For example, molecules of modulus I have 2 1 2 1 2 1 2 1 , modulus II, 2 2 1 1 2 2 1 1 , modulus, IV 2 2 2 2 1 1 1 1 . Upon the addition of monovalent alkaline cations or the introduction of the peptide solutions into physiological media, these oligopeptides spontaneously assemble to form macroscopic structures that can be fabricated into various geometric shapes [12,14]. Scanning EM reveals that the matrices are made of interwoven filaments that are about 10–20 nm in diameter and pores about 50–100 nm in diameter [7,9,14]. A variety of mammalian cell types have been shown to attach to the peptide matrices. These peptide materials can also have various biological properties by incorporating different biological ligands [9,12].
Reactive & Functional Polymers 41 (1999) 91–102 / locate / react
Peptide self-assembly in functional polymer science and engineering
Shuguang Zhang*, Michael Altman
1. Introduction Molecular self-assembly, by definition, is the spontaneous organization of molecules under thermodynamic equilibrium conditions into structurally well-defined arrangements due to noncovalent es undergo self-association forming hierarchical structures without external instruction. Molecular self-assembly is ubiquitous in nature and has recently emerged as a new strategy in chemical synthesis, polymer science and engineering [1].