Soil Carbon and Nitrogen Dynamics Following Application of Pig Slurry for the 19th
土壤碳氮磷生态化学计量特征及影响因素概述
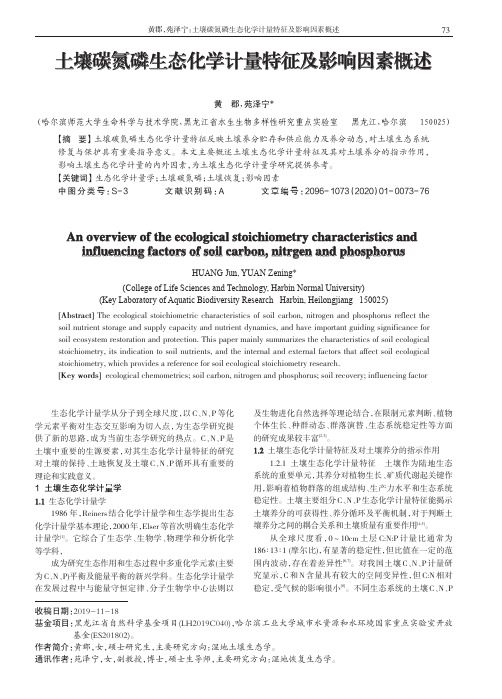
生态化学计量学从分子到全球尺度,以C、N、P 等化学元素平衡对生态交互影响为切入点,为生态学研究提供了新的思路,成为当前生态学研究的热点。
C、N、P 是土壤中重要的生源要素,对其生态化学计量特征的研究对土壤的保持、土地恢复及土壤C、N、P 循环具有重要的理论和实践意义。
1土壤生态化学计量学1.1生态化学计量学1986年,Reiners 结合化学计量学和生态学提出生态化学计量学基本理论,2000年,Elser 等首次明确生态化学计量学[1]。
它综合了生态学、生物学、物理学和分析化学等学科,成为研究生态作用和生态过程中多重化学元素(主要为C、N、P)平衡及能量平衡的新兴学科。
生态化学计量学在发展过程中与能量守恒定律、分子生物学中心法则以及生物进化自然选择等理论结合,在限制元素判断、植物个体生长、种群动态、群落演替、生态系统稳定性等方面的研究成果较丰富[2,3]。
1.2土壤生态化学计量特征及对土壤养分的指示作用1.2.1土壤生态化学计量特征土壤作为陆地生态系统的重要单元,其养分对植物生长、矿质代谢起关键作用,影响着植物群落的组成结构、生产力水平和生态系统稳定性。
土壤主要组分C、N、P 生态化学计量特征能揭示土壤养分的可获得性、养分循环及平衡机制,对于判断土壤养分之间的耦合关系和土壤质量有重要作用[4,5]。
从全球尺度看,0~10cm 土层C:N:P 计量比通常为186∶13∶1(摩尔比),有显著的稳定性,但比值在一定的范围内波动,存在着差异性[6,7]。
对我国土壤C、N、P 计量研究显示,C 和N 含量具有较大的空间变异性,但C:N 相对稳定,受气候的影响很小[8]。
不同生态系统的土壤C、N、P土壤碳氮磷生态化学计量特征及影响因素概述(哈尔滨师范大学生命科学与技术学院,黑龙江省水生生物多样性研究重点实验室黑龙江,哈尔滨150025)【摘要】土壤碳氮磷生态化学计量特征反映土壤养分贮存和供应能力及养分动态,对土壤生态系统修复与保护具有重要指导意义。
土壤含水率对埋地管道热力影响的数值模拟
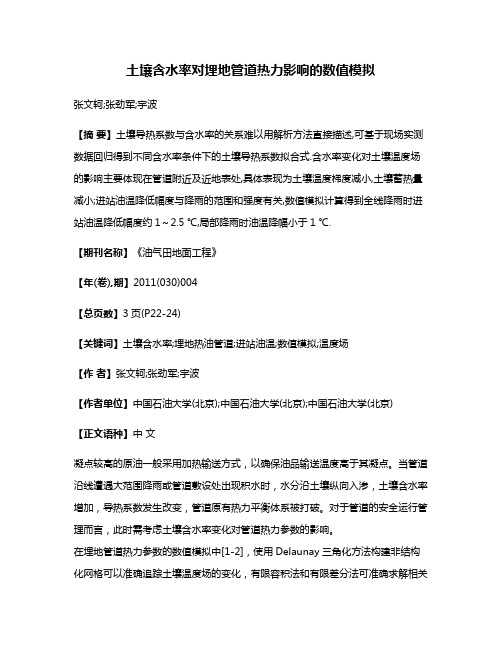
土壤含水率对埋地管道热力影响的数值模拟张文轲;张劲军;宇波【摘要】土壤导热系数与含水率的关系难以用解析方法直接描述,可基于现场实测数据回归得到不同含水率条件下的土壤导热系数拟合式.含水率变化对土壤温度场的影响主要体现在管道附近及近地表处,具体表现为土壤温度梯度减小,土壤蓄热量减小;进站油温降低幅度与降雨的范围和强度有关,数值模拟计算得到全线降雨时进站油温降低幅度约1~2.5 ℃,局部降雨时油温降幅小于1 ℃.【期刊名称】《油气田地面工程》【年(卷),期】2011(030)004【总页数】3页(P22-24)【关键词】土壤含水率;埋地热油管道;进站油温;数值模拟;温度场【作者】张文轲;张劲军;宇波【作者单位】中国石油大学(北京);中国石油大学(北京);中国石油大学(北京)【正文语种】中文凝点较高的原油一般采用加热输送方式,以确保油品输送温度高于其凝点。
当管道沿线遭遇大范围降雨或管道敷设处出现积水时,水分沿土壤纵向入渗,土壤含水率增加,导热系数发生改变,管道原有热力平衡体系被打破。
对于管道的安全运行管理而言,此时需考虑土壤含水率变化对管道热力参数的影响。
在埋地管道热力参数的数值模拟中[1-2],使用Delaunay三角化方法构建非结构化网格可以准确追踪土壤温度场的变化,有限容积法和有限差分法可准确求解相关水力、热力模型。
土壤导热系数与含水率的关系难以用解析方法直接描述,可基于现场实测数据回归得到不同含水率条件下的土壤导热系数拟合式。
土壤含水率变化时,导热系数会发生较大变化。
土壤含水率的变化主要需考虑两种情况,一是不同深度土壤含水率的差异,二是由于降雨、地表积水入渗引起的土壤含水率的上升。
东北地区土壤为亚黏土,在土壤纵向方向导热系数受含水率影响较大。
根据现场实测数据[3],不同深度处的土壤含水率可由以下拟合式得到式中w为土壤初始含水率(%);h为管道埋深(m)。
当管道沿线出现降雨或地表积水时,土壤含水率与积水深度、降雨量、降雨时间、土壤种类、孔隙度和有机物含量等因素有关。
土与基础结构动力相互作用的饱和弹性半空间理论

土与基础结构动力相互作用的饱和弹性半空间理论土与基础结构动力相互作用的饱和弹性半空间理论引言:土与基础结构的相互作用是土力学和地震工程领域中的重要研究课题。
在地震和其他动力荷载作用下,土体的动态特性对基础结构的动态响应和稳定性起着至关重要的作用。
本文将介绍土与基础结构动力相互作用的饱和弹性半空间理论,该理论基于弹性连续体力学和Biot动力响应理论,并考虑了饱和土的非均匀渗流效应。
1. 土弹性力学基础土体是一种多孔介质,具有弹性和连续性。
土体的弹性性质可以通过与岩石和金属类似的弹性力学理论来描述。
弹性体在受力时产生应变,并且当撤离力时能够完全恢复到无应变状态。
土体的弹性性质是通过弹性模量和泊松比来表征。
弹性模量是土体在单位应力作用下发生的应变,泊松比是侧向收缩应变与轴向应变之比。
2. 土与结构动力相互作用的Biot理论Biot理论是描述多孔弹性体动力响应的重要理论。
Biot理论考虑了土体的质量,弹性性质和渗流特性,并基于弹性连续体力学和一组渗流方程,提供了解析土体动力响应的框架。
该理论考虑了土体的质量能量平衡、线弹性力学和物质平衡方程。
3. 饱和弹性半空间模型饱和弹性半空间模型是一种简化的土体模型,它可以有效地描述土与基础结构之间的动力相互作用。
半空间指的是没有边界的无限土体模型。
饱和弹性半空间模型的基本假设是土体是均匀饱和、各向同性、弹性均一的介质,且无边界限制。
4. 动力相互作用分析方法饱和弹性半空间模型可以通过数值方法进行分析,例如有限元法和边界元法。
数值方法可以建立基于弹性理论和Biot动力响应理论的土体和结构的数学模型,通过求解模型的运动方程和边界条件来预测土体和结构的动力响应。
5. 非均匀渗流效应的考虑饱和土体中的渗流对土体的动力响应有着重要的影响。
由于渗流,土体中的孔隙水压强度会发生变化,从而改变土体弹性模量和阻尼特性。
非均匀渗流效应的考虑可以通过将渗流过程纳入动力相互作用分析中的渗流方程来完成。
间作对土壤水热·温室气体排放及作物的影响综述

间作对土壤水热㊃温室气体排放及作物的影响综述杨沛文,柴红敏,刘小梦,李昱儒,张又天,张镇企,陈可莹,李陆生∗㊀(华北水利水电大学,河南郑州450046)摘要㊀为了因地制宜地选择种植模式,充分利用水土资源㊁保护粮食安全㊁减少农田温室气体排放和促进我国绿色农业可持续发展,归纳了国内外关于间作系统的耗水量㊁土壤蒸发㊁土壤温度㊁温室气体排放㊁间作种间关系的影响因素及其对作物的影响㊂结果表明,间作模式的耗水量主要受环境因素与作物类型的影响,并且可以通过整合单作常用的水分调节措施减少耗水量,提高水分利用效率;间作具有双向调节土壤温度的功能;合理的间作模式可以改变田间小气候和土壤中微生物的生存环境,从而降低CO 2㊁N 2O 的排放,增加CH 4的吸收量;间作可以使水㊁肥在时空尺度上得到充分吸收,达到增加产量㊁改善品质的目的㊂关键词㊀间作;土壤水热;温室气体;种间关系;生长发育中图分类号㊀S 344.2㊀㊀文献标识码㊀A㊀㊀文章编号㊀0517-6611(2023)13-0008-06doi :10.3969/j.issn.0517-6611.2023.13.002㊀㊀㊀㊀㊀开放科学(资源服务)标识码(OSID):Review on the Effects of Intercropping on Soil Moisture and Heat ,Greenhouse Gas Emissions and CropsYANG Pei-wen ,CHAI Hong-min ,LIU Xiao-meng et al㊀(North China University of Water Resources and Electric Power,Zhengzhou,Henan 450046)Abstract ㊀The aims of this study are to select planting mode according to local conditions,make full use of water and soil resources,protect food security,reduce greenhouse gas emissions from farmland and promote sustainable development of green agriculture in China.The effects of intercropping on water consumption,soil evaporation,soil temperature,greenhouse gas emissions,factors affecting interspecific relationship of intercropping system and their effects on crops were reviewed both at home and abroad.The results show that the water consumption of inter-cropping mode is mainly affected by environmental factors and crop types.Water consumption can be reduced and water use efficiency can be improved by referring to common water regulation measures in monoculture.Intercropping has the function of bidirectional regulation of soil temperature ,and can change the field microclimate and the living environment of microorganisms in the soil,thus reducing the emission of CO 2,N 2O and CH 4.Intercropping can make water and fertilizer be fully absorbed in space-time scale to increase yield and improve quality.Key words ㊀Intercropping;Soil moisture and temperature;Greenhouse gas;Interspecific relationship;Growth and development作者简介㊀杨沛文(1998 ),男,河南新乡人,硕士研究生,研究方向:农业水资源高效利用㊂∗通信作者,讲师,博士,从事水土资源高效利用研究㊂收稿日期㊀2022-12-23㊀㊀当前,水资源短缺情况加剧㊁耕地面积日益缩减,使我国粮食安全问题面临严峻挑战,并且随着人口的增长以及对饲料㊁纤维和生物燃料的需求增加,预计到2050年粮食需求量会翻一番[1],供需关系不平衡使粮食安全问题更突出㊂温室效应也是当今重大的环境问题,会造成粮食减产和极端天气频繁等自然灾害,农业系统的温室气体排放在近十年增加了10.1%[2],并占全球温室气体总量的14.0%[3]㊂因此,面临资源与环境的多重挑战,需要选择一种高产高效且生态友好的农业发展方式来挖掘农业生产潜力,保障粮食安全,带动我国农业的绿色可持续发展㊂间作是提高农田生产能力㊁降低农业生产环境成本㊁推动我国绿色农业可持续发展的重要手段㊂其最早可追溯到西汉时期,在‘祀胜之书“中出现了有关瓜豆间作的记载㊂20世纪60年代,间作在我国得到了广泛推广,有粮饲间作㊁农林间作㊁林果间作㊁粮菜间作等多种类型,尤其玉米和豆科植物间作最为普遍[4]㊂间作的主要优势包括可以充分吸收利用土壤中的多余水分与养分[5],可以通过影响温室气体排放的关键因子来减少土壤温室气体的排放[6],可以充分利用光能㊁时间和空间资源来提高生产力[7]㊂所以发展间作对提高农业生产的经济效益㊁社会效益㊁环境效益㊁节约土地资源和保障粮食生产等具有重大现实意义㊂虽然国内外学者对间作条件下的土壤㊁作物与生态环境影响等方面做了较多研究,但缺乏系统的归纳应用于指导实践㊂因此,该研究综述间作系统的土壤水热㊁土壤温室气体排放以及作物生长发育特点,旨在为农业生产过程中选择合适的耕作方式,充分利用水土资源㊁降低温室气体排放㊁增加作物产量和改善作物品质,为我国绿色农业可持续发展提供参考㊂1㊀间作系统的土壤水热状况1.1㊀间作系统的耗水量㊀间作系统的耗水量主要受环境条件和作物类型的影响[8]㊂间作条带之间由于水分竞争,会存在大量水分运移[9],为了满足间作系统的高产,就需要足够的水分支撑,所以在水资源充足地区发展间作具有巨大潜力㊂了解间作系统耗水规律,将供水与作物耗水规律相结合可以有效降低间作群体耗水量,从而使水资源短缺地区的间作得以发展㊂当前对间作耗水量的研究主要集中在空间布局㊁灌溉制度㊁施肥制度㊁耕作方式和覆盖措施等方面[10]㊂减少耗水量的基础是合理的作物搭配与适当的灌溉施肥㊂从空间布局上看,徐鹏等[11]指出,间作耗水量随着棉花行数的增加和株距的减少显著增大,因为间作棉花可以充分利用土壤贮水,间作系统的耗水量比单作加权平均高9.5%㊂Wang 等[12]认为在小麦/玉米间作系统中,耗水量随着玉米种植密度的增加而减少,并指出玉米根长密度的增加,地下相互作用的增强,使得土壤蒸发降低,减少了无效耗水量;从灌溉制度上看,牛伊宁等[13]研究指出,玉米/豌豆间作系统降低10%灌水水平并没有对耗水量产生显著影响,适当降低灌水水平可以在不影响产量的情况下减少无效耗水量;从施肥制度上看,李倩倩等[14]通过小麦/玉米间作试验发现,氮肥的使用会显著增加作物的耗水量,李含婷等[15]指出减氮㊀㊀㊀安徽农业科学,J.Anhui Agric.Sci.2023,51(13):8-1325.0%施肥可以减少玉米/绿肥间作系统的整体耗水,这些研究表明可以通过施肥调节间作耗水量㊂也有研究认为间作系统的耗水量受氮肥影响不显著[16]㊂通过优化耕作方法和覆盖也可以减少耗水量㊂例如,在小麦/玉米间作中,小麦留茬收割并压倒覆盖与不留茬相比,耗水量减少了0.8%~6.3%[17]㊂垄作交替灌溉可使高㊁低灌溉水平的耗水量分别比传统灌溉降低5.3%㊁3.0%[18]㊂研究表明,通过整合单作中常用的水分调节措施,可以有效减少间作的耗水量㊂1.2㊀间作系统的土壤蒸发㊀土壤蒸发作为无效耗水量在农田总耗水中所占的比重较大㊂间作模式对土壤蒸发的影响较为复杂,其可以通过种间竞争和互补对土壤蒸发和土壤水分消耗的空间梯度产生影响㊂间作系统的土壤蒸发与单作相比,受到配对作物㊁种植空间与时间等多种因素的影响,明确影响间作土壤蒸发的关键因子,对减少无效耗水有重要的指导作用㊂柴强等[19]研究表明,间套作生育期较长,对于整个生育期土壤的总蒸发量大于单作,但是间作系统的日平均土壤蒸发量较低㊂刘浩等[20]指出,棵间土壤蒸发主要受表层土壤含水率和叶面积指数的影响,在返青前小麦/玉米间作和单作土壤蒸发差距不大,而返青后间作的土壤蒸发显著高于单作,因为单作小麦冠层覆盖率高,而间作的预留行裸漏导致无效的水分消耗㊂高阳等[21]通过玉米/大豆间作试验指出玉米条带对地面的覆盖度小于大豆,玉米ʒ大豆2ʒ3带型的土壤蒸发量大于1ʒ3带型㊂多年来,研究者提出了各种减少间作无效农田用水量的策略,通过优化灌溉水平㊁施肥方式㊁覆盖和耕作措施来减少土壤蒸发㊂交替灌溉可通过减少地表土壤含水率来减少土壤水分蒸发[18],氮肥后移有利于间作玉米在豌豆收获后的生长发育,增加地表覆盖度可使间作系统的土壤蒸发减少15%~30%[16],免耕和铺设地膜增加了土壤与大气之间水热交换的物理阻隔,阻断了交换途径从而降低土壤蒸发[22]㊂1.3㊀间作系统的土壤温度㊀土壤温度是植物生长的关键因素,也是评估间作功能的重要参数㊂适宜的土壤温度有助于土壤和大气之间的气体交换,增强土壤中的微生物和根系的活性,并且可以降低温室气体的排放[23]㊂土壤温度的变化不仅取决于大气温度的波动,还受到种植制度的影响㊂间作系统复杂的边界条件会影响其土壤温度的变化㊂间作可以抑制土壤温度的升高,在一些炎热的月份,间作叶面积指数较大的作物可以有效缓解高温胁迫对作物造成的损害㊂Ai等[24]进行了3年枣/棉间作试验,结果表明,与单作枣树相比,间作使枣树与棉花6月以后的土壤温度均下降㊂Nyawade等[25]研究认为,间作增加了地表覆盖率和土壤水分含量,提高了植物拦截辐射的能力,并显著降低了0~ 30cm土层的土壤温度㊂紫云英/油菜间作[26]和核桃/小麦间作[27]等也得到了间作在高温时段可以降低土壤温度的结论㊂间作也可以抑制土壤温度的降低,起到保温作用㊂Olasantan[28]研究得出,在间作系统中,低位作物阻碍了夜间土壤温度向高水平作物的消散㊂王来等[27]研究得出,核桃与小麦间作模式推迟了11月份土壤温度的下降,并指出是由于落叶覆盖地面起到了保温的作用,这种情况对冬小麦的生长有利㊂间作具有双向动态调节土壤温度的功能,因此间作的土壤温度稳定性显著高于单作㊂采取不同的间作模式,可以通过改变作物的冠层结构与生长情况使作物根系处于适宜的温度,促进作物生长㊂2㊀间作对温室气体排放的影响2.1㊀间作对CO2排放的影响㊀部分研究表明,间作可以降低CO2排放㊂从排放量上看,章莹等[29]指出,减量施氮处理下,甘蔗ʒ大豆1ʒ2间作CO2排放量较甘蔗单作显著降低35.58%㊂Wu等[30]通过小麦/板蓝根间作试验发现,间作处理的小麦生育期总CO2排放通量与小麦单作相比降低了29.3%㊂Qin等[31]通过3年的小麦/玉米间作和豌豆/玉米间作试验表明,2种间作模式的平均碳排放量分别比玉米单作降低32.0%和38.0%㊂从排放速率上看,曹永庆等[32]同时对3块试验田进行山稻/油茶间作试验发现,间作山稻使土壤呼吸速率比单作油茶分别降低54.6%㊁20.5%和13.8%,土壤呼吸受到抑制的关键原因是土壤含水率的下降㊂赵财等[33]测算对比了河西绿洲灌区不同种植模式下的土壤呼吸速率,指出不同间作模式下的土壤呼吸速率均小于单作玉米,其中小麦/玉米间作系统的土壤呼吸速率比玉米单作和小麦单作分别降低20.9%和26.3%㊂也有研究表明,间作并不会降低CO2的排放㊂常规施氮处理下甘蔗ʒ大豆1ʒ1间作模式与1ʒ2间作模式CO2排放总量较甘蔗单作CO2排放量均显著增加[29]㊂简忠领等[34]研究不同行距条件下玉米与白三叶草间作,认为土壤呼吸速率主要受行距的影响,受间作影响不显著㊂孟平等[35]对石榴/玉米间作试验发现,间作系统整个生育期的土壤呼吸速率平均值为3.0μmol/(m2㊃s),高于绿豆单作系统的平均值2.8μmol/(m2㊃s)㊂玉米/豇豆间作试验[36]和玉米/蚕豆间作试验[37]也得到了间作使CO2排放量增加的结论㊂综上,间作对土壤CO2的调控作用受作物种类㊁种植间距㊁施肥方式和试验时长的差异影响较大㊂对于间作是否具有减少CO2排放效果还存在争议㊂由于农业系统的稳定需要较长的时间,对农田CO2排放监控超过10年才能得出准确结论㊂2.2㊀间作对N2O排放的影响㊀农田土壤N2O排放量约占大气N2O排放总量的70.0%~90.0%[38]㊂氮肥的使用为土壤提供了充足的氮源,使N2O的排放量显著增加[39]㊂间作系统中,豆科植物的固氮作用可以为植物提供氮元素,所以研究者多研究包含豆科植物的间作系统对N2O排放的影响㊂间作豆科植物可以降低N2O的排放㊂Huang等[40]通过2年的间作试验得出,玉米与豆类间作可以有效降低N2O的排放量,其中玉米与大豆间作的减排幅度最大,2010与2011951卷13期㊀㊀㊀㊀㊀㊀㊀㊀㊀㊀㊀㊀㊀㊀杨沛文等㊀间作对土壤水热·温室气体排放及作物的影响综述年玉米/大豆间作的N2O排放量与玉米单作相比分别减少了25.5%与48.8%,并指出是由于间作使土壤含水量降低进而抑制了土壤N2O的排放㊂陈津赛等[41]研究发现,间作系统提高了土壤中的氮素吸收量,降低了土壤中微生物可利用的无机氮的含量,玉米/大豆间作的N2O排放量分别比单作玉米和单作大豆降低了36.7%和49.0%㊂但也有研究表明,间作并不会降低N2O的排放㊂Vachon[42]研究表明,玉米ʒ大豆1ʒ2和2ʒ3间作与单作系统土壤N2O累积排放量差异不显著㊂刘辉娟[43]指出,在整个生育期玉米/大豆间作的N2O排放量始终高于玉米单作,并且随着施氮量的增加,N2O排放量随之增大㊂甘蔗/大豆间作[29]和玉米/蚕豆间作试验[37]也得到了间作使N2O排放速率加快的结论,对比分析后发现,产生此结果的原因可能与取样方式方法㊁施肥方式㊁土壤性质㊁种植品种㊁种植比例以及不同地区气候不同有关㊂由此可见,N2O减排的主要原因是间作引起环境因子发生变化或者氮肥使用量的减少;增排的主要原因是间作环境在短时间内加快了土壤氮矿化速率㊂从长远来看,间作是否具有N2O增排效应还需对农田土壤长期监控㊂2.3㊀间作对CH4排放的影响㊀CH4的增温效果比CO2高28倍,在过去200年间,CH4的浓度持续增加[44]㊂甲烷的产生需要厌氧环境,因此旱作农田的CH4通常表现为吸收,研究表明每年旱地透气土壤可以吸收30t CH4[45]㊂增加旱作农田的吸收量,对环境保护有重要意义㊂间作可以促进土壤对CH4的吸收㊂尚小厦等[45]通过冬小麦/板蓝根间作试验得出,同等施肥条件下板蓝根/冬小麦间作比冬小麦单作CH4吸收量高34.0%,并推测是由于板蓝根的根系释放出的物质改变了土壤环境,对CH4的吸收产生影响㊂冬小麦与大蒜间作试验也得出了间作使土壤CH4吸收量增加的结论,并推测是由于间作模式改变了土壤中有关CH4菌群的活性[46]㊂也有研究表明,间作不利于CH4的吸收,沈亚文[6]进行了3年的玉米/大豆间作试验,发现间作CH4吸收速率均低于玉米和大豆单作㊂施肥可以对间作系统CH4的排放起调控作用㊂施肥不利于土壤对CH4的吸收,一方面是因为产CH4基质增加从而生产出更多的CH4,另一方面土壤中氮素的增加抑制土壤对CH4的氧化[45],可以在不影响总产量的前提下通过减少施肥量,来减少温室气体的排放[29]㊂也有研究表明施肥对CH4吸收没有明显影响[6]㊂合理的间作模式和施肥制度可以通过调节土壤环境,影响微生物的活性,增加CH4的吸收量㊂3㊀间作种间关系的影响因素及其对作物的影响3.1㊀间作种间关系的影响因素㊀间作条件下,种间促进和竞争同时对作物生长产生影响㊂物种间的促进作用可以通过提高土壤微生物的数量和酶的活性等来体现[47];竞争作用是由于有限资源的非比例共享或不同物种之间的相互影响使作物的生长受到抑制[48]㊂间作种间关系的影响因素包括作物组合㊁空间布局与环境因素㊂配对作物的选择是影响间作种间关系的首要因素㊂不同的作物具有不同的生物学特性,充分利用作物的特征以使间作系统的互补效应大于竞争㊂禾本科作物与豆科作物间作是一种典型的种植模式,豆科作物根系的固氮作用可以增加土壤肥力,同时禾本科作物根系分泌物可以促进豆科作物根系有关结瘤固氮基因的表达,提升豆科作物固氮作用[49]㊂深根系与浅根系作物搭配可以使土壤中的水分㊁养分得到高效利用,高秆作物与矮秆作物搭配可以在空间上使光能得到充分利用,生育期不同的作物搭配可以在时间上达到资源高效利用的目的㊂配对作物的选择有时可能与资源本身并不相关,麦/棉间作系统中小麦带为棉花提供了类似 防风带的屏障,很大程度地保障了棉农的收益[50]㊂所以,应在充分了解不同作物生长特性的基础上,因地制宜地发展间作模式,灵活利用不同形式的互补作用,以充分发挥间作的优势㊂适宜的空间布局是使间作模式的产量达到稳产㊁高产的基础㊂间作作物的空间布局包括配对作物的种植比例㊁株行距和共生期的长短㊂因间作的资源利用率高于单作,所以相同条件下间作的适宜的种植密度通常大于单作[51]㊂随着种植密度的增大,配对作物之间的种间关系也会随之改变[52]㊂过高的种植密度会加剧种内竞争,不利于作物生长;过低的种植密度会造成资源的浪费,达不到间作的目的㊂种间竞争的对象通常为水分㊁养分和光照等环境因子㊂作物所需资源是否充足往往影响着种间竞争的强弱[53],在竞争条件下其会优先发育获取限制性资源的器官,如玉米与其他作物间作会使玉米的根长㊁根体积㊁根表面积增加,以显著提高玉米吸收水分㊁养分的能力[54]㊂对限制性资源的竞争可以通过外部资源投入的方式来减弱,补充灌溉㊁氮肥后移等措施均已得到验证[16,18]㊂滴灌与其他灌溉措施相比具有高度可控性,精确控制不同作物在不同发育阶段的水分来调控作物生长,把滴灌引入间作模式可以达到调节种间关系的目的㊂3.2㊀间作对作物生长发育的影响㊀植株高度㊁叶面积指数和干物质积累动态可以直观反映作物的生长发育情况㊂玉米与大豆间作时,玉米的植株高度㊁叶面积指数和干物质积累量会得到增加,大豆的生长遭到抑制,增加大豆和玉米行距或者带距有利于促进这两种作物的生长发育[55]㊂艾鹏睿等[56]通过枣/棉间作试验发现,盆栽和大田间作条件下棉花的株高和叶面积指数在不同生育期均大于单作棉花㊂作物生长速度也是生长发育的一项重要指标,柏文恋等[57]通过对小麦/蚕豆间作研究发现,在小麦生长中后期,间作降低了小麦种内竞争压力,显著提高了小麦的生长速度㊂玉米与大豆间作时增加行距或带距可以使作物获得更大的生存空间,获取更多的光热资源,有利于缩短作物的生育期[58]㊂作物的生长发育情况要考虑地上和地下两部分㊂刘丽娟等[59]对玉米/木薯间作研究发现,木薯地上部分会由于受到遮阴而长高长细,地下部分木薯的根系不仅会促进玉米根系的生长,还使木薯根系氮磷钾的含量间作高于单作,可能01㊀㊀㊀㊀㊀㊀㊀㊀㊀㊀安徽农业科学㊀㊀㊀㊀㊀㊀㊀㊀㊀㊀㊀㊀㊀㊀㊀㊀㊀㊀㊀㊀㊀㊀㊀㊀㊀2023年是玉米根系呈上窄下宽分布,木薯根系呈上宽下窄分布使玉米和木薯根系构型形成互补,提高了养分利用效率,也可能是玉米与木薯根系之间的酶或微生物促进了养分的吸收㊂Jiao等[60]对玉米和花生间作研究发现,根系隔离开之后间作的产量和生物量优势分别降低了82.0%和67.0%,这表明玉米/花生间作系统的地下相互作用比地上相互作用更有利于间作作物的生长发育㊂在其他间作系统中也观察到相同的结果[61-62]㊂然而,在玉米/大豆套作系统中,地上相互作用比地下相互作用对作物生长发育的影响更大[63]㊂可能是花生/玉米间作比玉米/大豆间作有更长的共存期,根系形态和时空分布不同㊂也可能是限制作物生长的主导因素不同,水肥充足时,地上竞争会占主导地位㊂综上,作物生长发育因作物种类组合而异,并进一步受到土壤养分㊁种植间距等的影响㊂对间作系统进行优化,就必须充分了解地上和地下的相互作用在作物生长发育中的影响㊂3.3㊀间作对作物品质的影响㊀合理的间作模式可以提高作物的品质㊂方旭飞等[64]通过不同耕作模式下的玉米/大豆间作试验发现,间作可以提高玉米的粗蛋白和粗淀粉的含量,降低玉米的含水量和粗灰分含量,从而使玉米品质得到提高,其中地膜覆盖条件下玉米/大豆间作提高玉米品质的作用最明显㊂张向前等[65]通过对玉米与大豆㊁花生豆科植物间作研究发现,间作可以提高土壤中微生物的数量和酶的活性,无论施肥与不施肥间作均可以提高玉米籽粒蛋白质㊁赖氨酸和淀粉的含量㊂李美等[66]对不同比例玉米花生间作研究认为,间作时玉米的蛋白质和脂肪含量高于单作;花生由于受到遮阴作用影响其碳水化合物的积累,导致蛋白质含量高于单作,脂肪含量低于单作,调整间作比例可以使花生受到的不利影响减少㊂巩雪峰等[67]对茶树和松树间作发现,间作改善了茶园小气候,提高了土壤养分,茶叶的品质与单作茶园茶叶相比有明显提高㊂陈映彤等[68]指出辣椒/紫苏间作改变了群体结构的水㊁热㊁光以及土壤环境,从而显著提高了辣椒果实中的可溶性蛋白质㊁糖和V C的含量㊂刘天学等[69]对不同基因型玉米间作研究发现,不同的基因型玉米组合间作可以改善群体结构和利用杂交优势,达到提高产量和改善品质的目的㊂综上,通过调控耕作方式㊁作物品种㊁种植间距和水肥等因素,可以改变系统内环境和土壤环境,提高作物品质㊂3.4㊀间作对作物产量的影响㊀间作可以提高作物产量已被大量研究证实㊂例如,Li等[62]在玉米/豌豆间作研究中发现,玉米和豌豆的产量与相应单作相比均增加㊂间作模式下蚕豆是优势竞争者,具有产量优势,蚕豆收获后玉米获得了较长的恢复生长时间,并且蚕豆的根留在土壤中会使土壤中氮含量增加,使玉米增产㊂蔡倩等[7]对不同种植比例的玉米/大豆间作研究认为,玉米与大豆间作比例为2ʒ2㊁4ʒ4和6ʒ6时,间作系统中玉米均表现出产量优势,大豆均表现出产量劣势,玉米与豆科植物间作时,豆科植物产量降低主要是由于玉米对大豆产生遮光效应,可以通过选择耐阴的大豆品种,或者调节玉米/大豆条带间作系统中的冠层结构和茎秆特性来减少产量损失[70]㊂玉米/紫花苜蓿间作[71]和玉米/花生间作[72]也得到了间作会牺牲部分弱势作物的产量,来获得总产量优势的结论㊂也有研究发现,间作并没有明显产量优势㊂杨欢等[73]对玉米/花生间作研究得出土地当量比(LER)为0.89~ 1.13,主要原因是种间竞争不平衡,并且共生期较长,花生长期处于荫蔽状态下,虽然间作增加了养分吸收量但降低了利用效率㊂综上,间作产量受多种因素影响,并且对配对作物产量的影响结果不一致,在大部分情况下间作仍具有总产量优势㊂可以通过施肥㊁改变种植比例和间距和优化灌溉制度等使间作产量优势更加明显㊂3.5㊀间作对作物水分利用效率的影响㊀间作系统中,当其中一种作物处在生长旺期时,可以从另一条带吸收水分,利用两种作物的生态位的差异,可以提高水分利用效率[74]㊂刘斌等[75]指出,甜瓜/向日葵间作系统的水分效率比甜瓜单作和向日葵单作都有明显提高,高频少量灌水有利于提高间作系统的水分利用效率㊂李倩倩等[14]指出,小麦/玉米间作种植水分利用效率大于小麦和玉米单作,并且氮肥的使用提高了水分利用效率优势,一方面氮肥可以影响作物生长,增加地表覆盖面积,减少无效水分的消耗;另一方面合理添加氮肥可以通过增加产量,使水分利用效率提高㊂施肥对水分利用效率的影响与施用时期也有较大关系,滕园园等[16]对玉米和豌豆间作研究指出,氮肥后移可以使玉米在豌豆收获后迅速生长,提高间作系统冠层覆盖度,降低无效的水分消耗,提高水分利用率㊂水肥量要控制在合理范围,施肥量过高会造成徒长,籽粒灌浆减少[76];水肥量过低,会不利于作物的生长发育[71],降低水分利用效率㊂种植比例和间距直接影响水分的分布与利用㊂王照霞等[77]对4种带型结构的玉米豌豆间作研究发现,3ʒ3带型间作的水分利用效率最高且高于玉米和碗豆单作,是因为3ʒ3带型和2ʒ2带型中玉米所占比例高于3ʒ6带型和2ʒ4带型,玉米的高产量有利于提高水分利用效率;3ʒ3带型与2ʒ2带型相比具有较少的共生区,降低了豌豆与玉米的竞争㊂Rahman等[78]指出,不同带宽和不同宽窄行间距,可以影响间作系统的冠层覆盖度和叶面积指数,从而影响土壤蒸发㊁土壤含水量和水分利用效率㊂间作系统作物根系生长发育会对水分利用效率产生影响㊂张恩和等[79]对小麦/蚕豆间作研究表明,小麦与蚕豆根系生长的峰值时间不同,可以通过作物需水关键期的错位,使水肥得到充分的利用,从而提高水分利用效率㊂刘丽娟等[59]对玉米/木薯间作根系构型研究指出,玉米上窄下宽与木薯上宽下窄的根系形成互补,土壤上层的水分交替形成水分亏空带,再由土壤水势和根系提水作用使水分得到及时补充,使得间作水分利用效率高于单作㊂综上,间作有利于调配土壤中的水分,满足不同作物各1151卷13期㊀㊀㊀㊀㊀㊀㊀㊀㊀㊀㊀㊀㊀㊀杨沛文等㊀间作对土壤水热·温室气体排放及作物的影响综述。
Impact of nitrogen fertilization and soil tillage on arbuscular mycorrhizal fungal communities
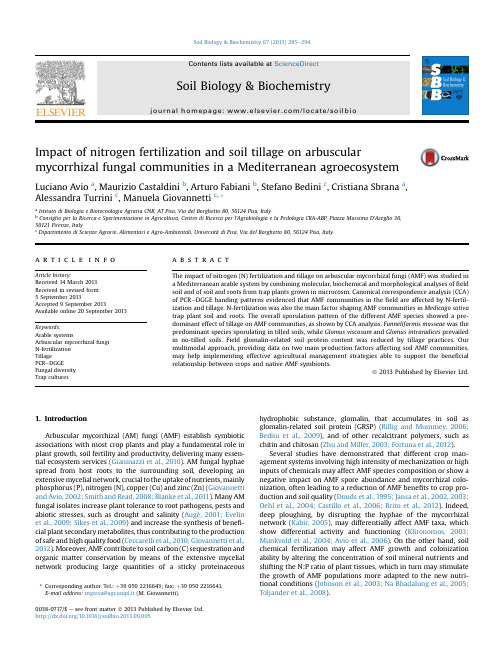
Impact of nitrogen fertilization and soil tillage on arbuscular mycorrhizal fungal communities in a Mediterranean agroecosystem Luciano Avio a,Maurizio Castaldini b,Arturo Fabiani b,Stefano Bedini c,Cristiana Sbrana a, Alessandra Turrini c,Manuela Giovannetti c,*a Istituto di Biologia e Biotecnologia Agraria CNR,AT Pisa,Via del Borghetto80,56124Pisa,Italyb Consiglio per la Ricerca e Sperimentazione in Agricoltura,Centro di Ricerca per l’Agrobiologia e la Pedologia CRA-ABP,Piazza Massimo D’Azeglio30, 50121Firenze,Italyc Dipartimento di Scienze Agrarie,Alimentari e Agro-Ambientali,Universitàdi Pisa,Via del Borghetto80,56124Pisa,Italya r t i c l e i n f oArticle history:Received14March2013Received in revised form5September2013Accepted9September2013 Available online20September2013Keywords:Arable systemsArbuscular mycorrhizal fungiN-fertilizationTillagePCR e DGGEFungal diversityTrap cultures a b s t r a c tThe impact of nitrogen(N)fertilization and tillage on arbuscular mycorrhizal fungi(AMF)was studied in a Mediterranean arable system by combining molecular,biochemical and morphological analyses offield soil and of soil and roots from trap plants grown in microcosm.Canonical correspondence analysis(CCA) of PCR e DGGE banding patterns evidenced that AMF communities in thefield are affected by N-fertil-ization and tillage.N-fertilization was also the main factor shaping AMF communities in Medicago sativa trap plant soil and roots.The overall sporulation pattern of the different AMF species showed a pre-dominant effect of tillage on AMF communities,as shown by CCA analysis.Funneliformis mosseae was the predominant species sporulating in tilled soils,while Glomus viscosum and Glomus intraradices prevailed in no-tilled soils.Field glomalin-related soil protein content was reduced by tillage practices.Our multimodal approach,providing data on two main production factors affecting soil AMF communities, may help implementing effective agricultural management strategies able to support the beneficial relationship between crops and native AMF symbionts.Ó2013Published by Elsevier Ltd.1.IntroductionArbuscular mycorrhizal(AM)fungi(AMF)establish symbiotic associations with most crop plants and play a fundamental role in plant growth,soil fertility and productivity,delivering many essen-tial ecosystem services(Gianinazzi et al.,2010).AM fungal hyphae spread from host roots to the surrounding soil,developing an extensive mycelial network,crucial to the uptake of nutrients,mainly phosphorus(P),nitrogen(N),copper(Cu)and zinc(Zn)(Giovannetti and Avio,2002;Smith and Read,2008;Blanke et al.,2011).Many AM fungal isolates increase plant tolerance to root pathogens,pests and abiotic stresses,such as drought and salinity(Augé,2001;Evelin et al.,2009;Sikes et al.,2009)and increase the synthesis of benefi-cial plant secondary metabolites,thus contributing to the production of safe and high quality food(Ceccarelli et al.,2010;Giovannetti et al., 2012).Moreover,AMF contribute to soil carbon(C)sequestration and organic matter conservation by means of the extensive mycelial network producing large quantities of a sticky proteinaceous hydrophobic substance,glomalin,that accumulates in soil as glomalin-related soil protein(GRSP)(Rillig and Mummey,2006; Bedini et al.,2009),and of other recalcitrant polymers,such as chitin and chitosan(Zhu and Miller,2003;Fortuna et al.,2012).Several studies have demonstrated that different crop man-agement systems involving high intensity of mechanization or high inputs of chemicals may affect AMF species composition or show a negative impact on AMF spore abundance and mycorrhizal colo-nization,often leading to a reduction of AMF benefits to crop pro-duction and soil quality(Douds et al.,1995;Jansa et al.,2002,2003; Oehl et al.,2004;Castillo et al.,2006;Brito et al.,2012).Indeed, deep ploughing,by disrupting the hyphae of the mycorrhizal network(Kabir,2005),may differentially affect AMF taxa,which show differential activity and functioning(Klironomos,2003; Munkvold et al.,2004;Avio et al.,2006).On the other hand,soil chemical fertilization may affect AMF growth and colonization ability by altering the concentration of soil mineral nutrients and shifting the N:P ratio of plant tissues,which in turn may stimulate the growth of AMF populations more adapted to the new nutri-tional conditions(Johnson et al.,2003;Na Bhadalung et al.,2005; Toljander et al.,2008).*Corresponding author.Tel.:þ390502216643;fax:þ390502216641.E-mail address:mgiova@agr.unipi.it(M.Giovannetti).Contents lists available at ScienceDirectSoil Biology&Biochemistry journal h omepage:www.elsevier.co m/lo cate/soilbio0038-0717/$e see front matterÓ2013Published by Elsevier Ltd./10.1016/j.soilbio.2013.09.005Soil Biology&Biochemistry67(2013)285e294The data available on the impact of different levels of tillage and chemical fertilization on AMF community composition and dy-namics indicate that such major production factors should be tested in dedicated experimental arable systems,in order to reach a better understanding of the driving forces that shape AM fungal communities(Moebius-Clune et al.,2013a)and to implement effective agricultural management strategies supporting crop plant-beneficial soil microorganisms.A comprehensive and exhaustive evaluation of changes in AMF community diversity produced by anthropogenic and environmental variables may be difficult to accomplish utilising singular approaches.Actually, morphological analyses based on spores collected in thefield may miss non sporulating species or those represented by old and parasitized spores,while root DNA analyses may reveal only the amplifiable DNA,representing a subset of AMF communities colo-nizing the sampled roots,which may differ from those detected in rhizosphere or bulk soil and from those described using morpho-logical analyses as well(Hempel et al.,2007;Cesaro et al.,2008; Mirás-Avalos et al.,2011).The aim of the present study was to evaluate the impact of N-fertilization and tillage on AMF abundance and diversity,focussing on a long-term experimental site in a Mediterranean arable system. To this aim,we combined molecular,biochemical and morpho-logical analyses to assess:i)AMF diversity infield soil,by means of polymerase chain reaction(PCR)e denaturating gradient gel elec-trophoresis(DGGE)analysis of18S rRNA gene fragments,a mo-lecularfingerprinting technique widely used to detect the modifications induced by different factors on soil microbes(Smalla et al.,2001;Castaldini et al.,2005;Oliveira et al.,2009);ii)AMF abundance and diversity,by means of morphological and molecular identification of spores produced in trap plants grown in micro-cosm,a technique providing newly produced spores suitable for morphological identification(Oehl et al.,2003,2004;Yao et al., 2010);iii)AMF diversity in soil and roots of trap plants,by means of PCR e DGGE analysis of18S rRNA gene fragments;iv)GRSP con-tent infield and trap cultures soil.2.Materials and methods2.1.Study site and soil samplingThe study was conducted at the“Pasquale Rosati”experimental farm near Agugliano,Italy(43 320N,13 220E,100m a.s.l.,slope10%). The soil is a calcaric gleyic cambisol almost free of gravel,with a high clay and calcium content.The climate is dry-summer subtropical (Mediterranean),with a mean annual rainfall in the period1998e 2008of786mm.The highest mean monthly temperature(30.6 C) and the lowest precipitation(35mm)occurred in July.The lowest mean monthly temperature(3.0 C)occurred in January and the highest precipitation(105mm)in September(De Sanctis et al.,2012). The experimental site belong to a long-term tillage experiment, established in1994,with a two year rotation of maize(Zea mays L.) and durum wheat(Triticum durum L.)since2002,and designed as a split plot with tillage treatments assigned to the main plots(each 1500m2in size)and N-fertilization treatments assigned to subplots (each500m2in size).The experiment was replicated in two blocks with treatments repeated in the same plots every year.In the present study,soil sampling was performed in the subplots treated with no N-fertilization(0)and90kg haÀ1N(90)as ammonium nitrate,both in the conventional tillage(CT)and in the no tillage(NT)treatment. CT treatment consisted of ploughing at a depth of40cm and double harrowing before sowing,whereas NT plots were left undisturbed except for sod seeding,crop residuals and weed chopping and total herbicide spraying prior to seeding.For data on crop yield and soil characterisation,see De Sanctis et al.(2012).After wheat harvest the experimental area was sampled in Autumn2006by randomly collecting four15cm deep soil cores from each of the eight subplots.The four soil cores were pooled to obtain samples of about2.0kg which were air-dried and stored at 4 C until processed.Two hundred grams of each sample were used for GRSP analysis and the remaining soil for establishment of trap cultures.Forfield soil DNA analysis,soil samples(three replicates) were taken from one subplot of the four relevant treatments,for a total of twelve samples,then stored atÀ20 C until processed.2.2.Trap cultures and spore analysisEach soil sample was mixed,1:1by volume,with Terragreen (calcined attapulgite clay,Oil Dri,Chicago,IL),and poured into four 750cm3plastic pots,two for each of the two trap plant species utilized,Z.mays and Medicago sativa L.Plants were grown in glasshouse,under ambient natural light and temperature condi-tions and supplied with tap water as needed.In addition,they received weekly fertilization with half strength Hoagland’s solution (10mL per pot).After six months’growth,three soil samples(10g each)were collected from each pot and processed.AMF spores and sporocarps were extracted by wet-sieving and decanting,using a set of nested sieves,down to a mesh size of50m m(Gerdemann and Nicolson,1963),thenflushed into Petri dishes and examined under a dissecting microscope(Wild,Leica,Milano,Italy).The spores were separated into groups,according to their morphology.Spores were isolated by using capillary pipettes,mounted on microscope slides in polyvinyl alcohol lacto-glycerol(PVLG)and in PVLGþMelzer’s reagent(1:1,v:v)and examined under a Polyvar light microscope (Reichert-Young,Vienna,Austria).Qualitative spore traits(spore shape,colour and size,spore wall structure and shape,colour and size of the subtending hypha)were examined on at least50spores for each morphotype.Morphotype identifications were based on original descriptions and current species descriptions available online(International Culture Collection of(Vesicular)Arbuscular Mycorrhizal Fungi[/fungi/taxonomy/ speciesID.htm];Prof.Janusz Blaszkowski website at Szczecin University[.pl/Glomeromycota/]).Since important changes of AMF nomenclature have been recently pro-posed by different authors(Oehl et al.,2011;Krüger et al.,2012), with some taxa inconsistently named,we utilized the new bi-nomials for consistent names and maintained the previous ones for the others.After sixteen months’growth,three soil samples were collected from each pot and processed as described above,with the aim of retrieving also late sporulating AMF species(Oehl et al.,2009).The data reported are from such a sampling.2.3.Field soil DNA extractionDNA extraction was performed on500mg of eachfield soil sample,with the FastDNAÒSpin Kit for Soil(MP Biomedicals,Solon, OH)according to manufacturer’s instructions,with minor modifi-cations:a double homogenization in the FastPrepÒInstrument(MP Biomedicals)for30s at a speed setting of6.0and25s at a speed setting of6.5,and afinal resuspension in100m L of TE buffer(10mM Tris e HCl,0.1mM EDTA pH8).The DNA was then purified with the DNA Clean Up Spin Kit(GENOMED GmbH,Löhne,Germany),ac-cording to manufacturer’s instructions.2.4.DNA extraction from roots and soil of trap culturesSoil and roots of Z.mays and M.sativa were collected from trap cultures six months after establishment.Three samples of roots (100mg)and soil(500mg)were utilized for each plant species andL.Avio et al./Soil Biology&Biochemistry67(2013)285e294 286treatment.Root DNA was extracted in liquid nitrogen using DNeasy Plant Mini Kit(QIAGEN GmbH,Hilden,Germany),according to the manufacturer’s protocol.Soil DNA was extracted as described above.2.5.DNA extraction from sporesIntact,healthy spores belonging to the following morphospecies were isolated from trap cultures six months after establishment and utilized for DNA extraction:a)Glomus viscosum T.H.Nicolson (pools of spores);b)Glomus intraradices N.C.Schenk&G.S.Sm. (pools of spores);c)Funneliformis mosseae(T.H.Nicolson&Gerd.)C. Walker&A.Schüssler(single spores and sporocarps).Spores and sporocarps were manually collected with a capillary pipette under the dissecting microscope and cleaned by sonication(120s)in a B-1210cleaner(Branson Ultrasonics,Soest,NL).After three rinses in sterile distilled water(SDW),spores and sporocarps were surface sterilized with2%Chloramine T supplemented with streptomycin (400m g mLÀ1)for20min and rinsedfive times in SDW.Spore clusters,spores and sporocarps were selected under the dissecting microscope and transferred in Eppendorf tubes before DNA extraction(Redecker et al.,1997).2.6.DNA amplificationAliquots of soil DNA(50ng)were used to amplify the V3e V4 region of18S rDNA using the universal eukaryotic NS31GC primer (Kowalchuk et al.,2002)and the AM1primer(Helgason et al.,1998) in a50m L PCR mix consisting of250m M each primer,250m M each dNTP,1.5mM MgCl2,1ÂBuffer(67mM Tris e HCl pH8.8;16.6mM (NH4)2SO4;0.01%Tween-20)and2.5U of Taq DNA Polymerase (Polymed,Firenze,Italy).The reaction was performed in a iCycler thermal cycler(Bio-Rad Laboratories Inc.,Hercules,CA)with a protocol consisting of an initial cycle of95 C for3min,followed by 35cycles of94 C for30s,62.3 C for45s and72 C for60s,and a final extension step at72 C for7min.Each sample was amplified three times and the amplicons were pooled together before DGGE analysis.Root and fungal spore DNA amplifications were performed in the same conditions,except for the starting material(25ng),and for annealing time of spore samples(60s).2.7.Double gradient DGGE analysis of AMF communitiesThe analysis was performed with the INGENYphorUÒsystem (Ingeny International BV,Goes,The Netherlands)on a5e6%poly-acrylamide gel(acrylamide/bis37.5:1),under denaturation condi-tions(urea,7M;40%formamide with a denaturing gradient ranging from25to50%);the gels were run in1ÂTAE buffer at75V for17h at60 C and were stained with14mL of1ÂTAE containing 1.4m L of SYBRÒGold(Molecular Probes,Inc.,Eugene,OR)(dilution 1:10,000)for30min in the dark.Visualization and digital pictures were performed with a ChemiDoc System(Bio-Rad Laboratories). Using electrophoretic patterns,a matrix of the presence and absence of bands was obtained by GelCompar II 4.6software (Applied Maths NV,Sint-Martens-Latem,Belgium).2.8.Cloning and sequencing of18S rDNA fragmentsSelected PCR e DGGE bands pertaining to spores or roots samples were excised from the gel,resuspended in30m L of sterile TE and stored atÀ30 C.The DNAs extracted from the DGGE bands were re-amplified with the primers NS31GC and AM1and the PCR products were loaded onto a new DGGE gel to ensure the purity of each single band.The amplicons were then cloned into a pCRÒ4-TOPOÒvector using TOPO TA CloningÒkit for Sequencing(Invitrogen Corporation, Carlsbad,CA)and sequenced using the M13primer.Sequencing was carried out at the C.I.B.I.A.C.I.(University of Florence)using the ABI PRISMÒBigDyeÒTerminator v1.1Cycle Sequencing Kit(Applied Biosystems,Foster City,CA)according to the manufacturer’s recommendations.The parameters for cycle sequencing in the thermocycler Primus96plus(MWG Biotech, Ebersberg,D)were18s delay at96 C,followed by25cycles with 18s at96 C,5s at50 C and4min at60 C.Electrophoresis was performed on an ABI Prism310CE system(Applied Biosystems).2.9.Phylogenetic analysisSequences were entered in the BLASTn program of National Center for Biotechnology Information GenBank database(http:// /)to search for closely related sequences. Before phylogenetic analysis,sequences were screened with Chimera Check version2.7(Cole et al.,2003)(http://rdp.cme.msu. edu)and aligned with ClustalW program(Chenna et al.,2003), using Glomeromycota sequences available in GenBank.The phylogenetic tree was inferred by neighbour joining(NJ)method using Kimura2-parameter in TREECON for Windows software(Van de Peer and De Wachter,1994).The confidence of branching was assessed using1000bootstrap resamplings.The sequences were deposited at EMBL Nucleotide Sequence Database(/ embl/)under the accession numbers HE806381e HE806417.2.10.GRSP analyses offield and trap culture soilGRSP was extracted from soil using the procedures described by Wright and Upadhyaya(1996)for easily extractable(EE-GRSP)and total(T-GRSP)GRSP.EE-GRSP analyses were carried out onfield soil and on six months’old trap cultures.Briefly,EE-GRSP was extracted from1g of2mm-sieved soil with8mL of a20mM citrate solution, pH7.0,by autoclaving at121 C for30min.T-GRSP was extracted from1g of2mm-sieved soil samples,by repeated cycles with 50mM citrate,pH8.0,by autoclaving at121 C for60min.Ex-tractions of samples continued until the supernatant content of GRSP was under method detection limits(2mg mLÀ1).Superna-tants from each cycle were collected after centrifugation at10,000g for10min to pellet soil particles,pooled and stored at4 C until analysed.Protein content was determined by Bradford assay (Sigma e Aldrich,Inc.)with bovine serum albumin as the standard. Each determination was repeated three times.2.11.Statistical analysisData of spore counts and GRSP concentrations were analysed on IBM SPSS19.0software(SPSS Inc.,Chicago,IL).The GLM Univariate procedure was utilized to investigate the effects of tillage man-agement,fertilization levels,and host plants in trap cultures,as fixed factors,and their interactions,with block as random factor. Canonical correspondence analysis(CCA)was performed by using PAST1.99software(Hammer et al.,2001),on the presence/absence matrix based on DGGE banding pattern and on spore numbers after logarithmic transformation.Permutation test(n¼1000)was per-formed by using PAST software.3.Results3.1.PCR e DGGE analyses of AM fungal diversity infield soil and trap culturesCCA revealed a significant effect of N-fertilization on AMF communities of thefield plots(P¼0.007).Thefirst canonical axisL.Avio et al./Soil Biology&Biochemistry67(2013)285e294287explained 86.2%of the cumulative variance of PCR e DGGE banding patterns data,and the second one explained the remaining 13.8%(Fig.1).CCA showed an additional effect of tillage on AMF com-munity diversity (Fig.1).PCR e DGGE pro files of AMF communities occurring in the soil of trap cultures from different treatments were always more dissim-ilar than pro files from the same treatment (Fig.2).CCA suggests a separation of AMF soil (0N)communities of M.sativa and Z.mays trap plants,though not statistically signi ficant (P ¼0.172)(Fig.3).N-fertilization was the main factor affecting AMF communities occurring in M.sativa trap soil,as revealed by CCA of the relevant PCR e DGGE pro files,showing a clear-cut separation between pat-terns obtained from N-fertilized and unfertilized soil (P ¼0.009)(Fig.4A).A minor effect of tillage treatments was found (Fig.4A).The first canonical axis explained 90.1%of the cumulative variance of PCR e DGGE banding patterns data,and the second one explained the remaining 9.9%(Fig.4A).Consistent results were obtained by CCA of AMF communities occurring in M.sativa roots (P ¼0.002)(Fig.4B).3.2.Analyses of DNA sequencesNS31-GC/AM1amplicons obtained from plant roots,spores and sporocarps of trap cultures of unfertilized plots generated multiple PCR e DGGE bands,which,after excision from the gel,cloning and-1-0.500.511.52-1.8-1.4-1-0.6-0.20.20.611.41.8A x i s 2 (13.8%)Axis 1 (86.2%)CT0CT90NT0NT90TillageFertilizationCT0CT0NT0NT0NT90NT90CT90CT90Fig.1.Canonical correspondenceanalysis (CCA)biplot of V3e V4region of nuclear 18S rDNA PCR e DGGE fragments from three replicates of field soil from conventionally tilled (CT)and no tilled (NT)plots fertilized with 0(NT0,CT0)or 90(NT90,CT90)kg ha À1N.Fig.2.PCR e DGGE pro files of V3e V4region of nuclear 18S rDNA fragments from roots of Medicago sativa (M)trap cultures from conventionally tilled (CT)and no tilled (NT)plots fertilized with 0(NT0,CT0)or 90(NT90,CT90)kg ha À1N.-0.8-0.6-0.4-0.20.00.20.40.60.81.0-0.7-0.5-0.3-0.10.10.30.50.7A x i s 2 (0.9%)Axis 1 (99.1%)NT0MNT0ZCT0MCT0Z TillageHostNT0MNT0MCT0MCT0M CT0ZCT0ZNT0ZNT0ZFig.3.Canonical correspondence analysis (CCA)biplot of V3e V4region of nuclear 18S rDNA PCR e DGGE fragments from three replicates of trap culture soil from unfertilized no tilled (NT0)and conventionally tilled (CT0)plots,with Medicago sativa (M)and Zea mays (Z)as host plants.-1-0.8-0.6-0.4-0.200.20.40.60.8-1.2-0.8-0.400.40.8 1.2A x i s 2 (9.9%)Axis 1 (90.1%)NT90NT90NT90NT0NT0NT0CT0CT0CT0CT90CT90CT90TillageFertilizationA-1-0.8-0.6-0.4-0.200.20.40.60.811.2-1.4-1.0-0.6-0.20.20.6 1.01.4A x i s 2 (7.0%)Axis 1 (93.0%)NT0NT0NT0CT0CT0CT0CT90CT90CT90NT90NT90NT90TillageFertilizationBFig.4.Canonical correspondence analysis (CCA)biplot of V3e V4region of nuclear 18S rDNA PCR e DGGE fragments from (A)soil and (B)roots of Medicago sativa (M)trap cultures from conventionally tilled (CT)and no tilled (NT)plots fertilized with 0(NT0,CT0)or 90(NT90,CT90)kg ha À1N.L.Avio et al./Soil Biology &Biochemistry 67(2013)285e 294288sequencing,yielded a total of 37sequences with high similarity (98e 100%identity)to those of Glomeromycota,after BLASTn searches in GenBank databases.Only two sequences matched with Ascomycota sequences.PCR e DGGE bands obtained from M.sativa and Z.mays roots yielded 20sequences which grouped into four Glomeromycota sequence types,showing identities with sequences of both cultured and uncultured AMF deposited in GenBank databases.In particular,we recovered two sequence types,clustering with sequences of F.mosseae (Ag1sequence type)and G.intraradices /Glomus fas-ciculatum (Thaxt.)Gerd.&Trappe/Glomus irregulare B 1aszk.,Wubet,Renker &Buscot group,hereafter G.intraradices (Ag3sequenceFig.5.Neighbour-joining phylogenetic tree of glomeromycotan sequences derived from PCR e DGGE bands obtained from Medicago sativa and Zea mays trap plants.The analysis is based on V3e V4region of nuclear 18S rDNA sequences,and the tree is rooted with a reference sequence of Geosiphon pyriformis (X86686).Bootstrap values (>70%)were determined for neighbour joining (1000resamplings).Different sequence types are indicated in brackets:Ag1,Agugliano1;Ag2,Agugliano2;Ag3,Agugliano3;Ag4,Agugliano4;Ag5,Agugliano5.Sequences obtained in the present study are shown in bold with their accession numbers (HE806381e HE806417)followed by their DNA source (spores,spo-rocarps,roots)and treatment (trap cultures from conventionally tilled (CT)and no tilled (NT)plots fertilized with 0(NT0,CT0)kg ha À1N),with Medicago sativa (M)or Zea mays (Z)as trap plant.L.Avio et al./Soil Biology &Biochemistry 67(2013)285e 294289type)(Fig.5).Two other sequence types,Ag4and Ag5,which matched (99%identity)with sequences of uncultured Glomus species already present in GenBank were found (Table 1).Ag1and Ag3sequences were retrieved from all Z .mays and M.sativa roots,with the exception of M.sativa roots of NT0trap cultures,where Ag1was absent.Ag5sequences were retrieved from all trap cul-tures roots,while Ag4sequence type were found only in M.sativa roots,irrespective of the treatment (Fig.5).No sequences of G.viscosum were retrieved from trap plant roots.Blast and phylogenetic analyses of sequences derived from the ampli fication of spores and sporocarps lead to the identi fication of three separate clusters,Ag1(11sequences),Ag2(4sequences)and Ag3(2sequences),corresponding to F .mosseae ,G.viscosum and G.intraradices ,respectively (Fig.5,Table 1).3.3.Abundance and diversity of AMF spores produced in trap culturesThe numbers of AMF spores produced in trap cultures were consistently decreased by tillage in both M.sativa and Z.mays host plants (Fig.6),ranging from 35to 130and from 3to 34per 10g of soil,in no-tilled and tilled soil,respectively.AMF spore number was also affected by trap plant species,while a strong interaction (P <0.001)was found between host plant species and tillage/fertilization treatments.Therefore,distinct statistical analyses were performed for each host plant,which showed that in M.sativa spore production was marginally affected by tillage and fertilization treatments,while in Z.mays tillage signi ficantly decreased sporu-lation (Table 2).Moreover,an interaction between fertilization and tillage was detected (P ¼0.01).The overall sporulation pattern of the different AMF species showed a predominant effect of tillage,compared with that of host and fertilization treatments,as revealed by CCA (P ¼0.032).The first canonical axis explained 95.9%of the cumulative variance ofPCR e DGGE banding patterns data,and the second one explained the remaining 4.1%(Fig.7).F.mosseae was the predominant species sporulating in tilled soils,while G.viscosum and G.intraradices prevailed in no-tilled soils (Fig.8).Interestingly,G.intraradices spores were not retrieved from all tilled treatments.A low number of Funneliformis geosporus spores (T.H.Nicolson &Gerd.)C.Walker &A.Schüssler was retrieved only from M.sativa traps (Fig.8).With M.sativa as host plant,the number of G.viscosum spores was signi ficantly lower in trap cultures from tilled than from no-tilled soils (P ¼0.01),while F.mosseae spore number signi ficantly decreased in fertilized soil (P ¼0.001).With Z.mays as host plant,only G.viscosum spore number was signi ficantly affected by fertilization treatments (P ¼0.011),with a strong fertilization by tillage interaction (P ¼0.002),as a result of its high sporulation in fertilized and NT plots.3.4.GRSP content in field soil and in trap culturesBoth T-and EE-GRSP concentrations were larger in no-tilled than in tilled field soil,and correlated well each other (Pearson correlation ¼0.823;P <0.001).T-GRSP content was signi ficantly affected by tillage (P ¼0.023),and was about 36.1%larger in NT than in CT plots (Fig.9).On the other hand,fertilization did not affect GRSP content (P ¼0.132and 0.082,respectively for T-GRSP and EE-GRSP).No differences in GRSP content of trap culture soil were found.4.Discussion4.1.PCR e DGGE pattern analysis of AM fungal diversity in field soil and trap culturesCCA of PCR e DGGE pro files clearly showed that AMF commu-nities were affected by N-fertilization both in field soil and in roots and soil of M.sativa trap plants.Such findings are in agreement with a previous DGGE-based study showing differences in the community composition of AMF colonizing the roots of Festuca pratensis and Achillea millefolium in a Swedish grazed grassland along a gradient of soil N and P concentration (Santos et al.,2006).Other studies,performed on AMF spores,indirectly evidenced that AMF may be affected by the use of chemical fertilizers:for example Oehl et al.(2004)showed that organic farming,where the use of chemical fertilizers is not allowed,promoted higher AMF diversity and abundance than conventional agriculture,whilst other authors found a lower AMF diversity and abundance in N fertilised agro-ecosystems (Egerton-Warburton and Allen,2000).CCA of PCR e DGGE pro files further displayed an impact of tillage on AMF communities,supporting recent data obtained in long-term experiments in temperate regions (Mirás-Avalos et al.,2011;Mathew et al.,2012).Actually in our study the occurrence of weeds as a consequence of no-tillage and N fertilization (De Sanctis et al.,2012)may have represented a further factor affecting the abundance and diversity of AMF.However,the effects of tillage treatments on AMF communities observed in field soil were con firmed by CCA of PCR e DGGE pro files from roots and soil of M.sativa and Z.mays trap plants.In this work,CCA evidenced an effect of M.sativa and Z.mays trap plants on AMF soil communities and a major effect of N-fertilization on AMF occurring in the soil and in the roots of M.sativa plants,suggesting that the responses of AMF to different agronomical treatments may depend also by host plant taxon or its nutritional status (Giovannetti et al.,1988;Egerton-Warburton and Allen,2000).Indeed,Oliveira et al.(2009)showed that tropical maize genotypes contrasting for phosphorus ef ficiency had a greater in fluence on AMF rhizosphere community than the level of P in the soil.Table 1Nuclear SSU rDNA sequence types obtained from clones of DGGE excised bands of Medicago sativa and Zea mays roots and spores of AMF produced in trap cultures.Sequence types Identity (%)Taxonomic af filiation Ag1NG017178(100)Funneliformis mosseae Ag2AJ505813(99)Glomus viscosum Ag3AJ536822(99)Glomus intraradices Ag4GU353916(99)Uncultured Glomus sp.Ag5GU353731(99)Uncultured Glomus sp.20406080100120140160180NT0MNT90MNT0ZNT90ZCT0MCT90MCT0ZCT90ZS p o r e n u m b e r 10 g s o i lFig.6.Total AMF spore density in trap cultures from conventionally tilled (CT)and no tilled (NT)plots fertilized with 0(NT0,CT0)or 90(NT90,CT90)kg ha À1N,and with Medicago sativa (M)or Zea mays (Z)as trap plant,after sixteen months ’growth.Error bars refer to standard error of the means (n ¼2).L.Avio et al./Soil Biology &Biochemistry 67(2013)285e 294290。
大学英语2笔记
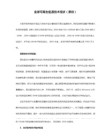
福师《大学英语(2)》第一课 Nature 课堂笔记Air Pollution空气污染Air supplies us with oxygen which is essential for our bodies to live.①Air is 99.9% nitrogen, oxygen, water vapor and inert gases.②Human activities can release substances into the air, some of which can cause problems for humans, plants, and animals.③空气为我们提供生存所必不可少的氧气。
空气中99.9 % 由氮气﹑氧气﹑水蒸气和惰性气体组成。
人类活动释放出的物质进入空气,其中的一些会对人类﹑植物和动物造成影响。
There are several main types of pollution and well-known effects of pollution which are commonly discussed. These include smog, acid rain, the greenhouse effect, and "holes" in the ozone layer.④Each of these problems has serious effects on our health and well-being as well as on the whole environment.污染有几大类型,所造成的影响广为人知,也经常引发讨论, 包括烟尘﹑酸雨﹑温室效应和臭氧层“空洞”。
这些问题已严重影响到我们的健康和幸福,也影响到整个环境。
One type of air pollution is the release of particles into the air from burning fuel for energy. Diesel smoke is a good example of this particular matter. This type of pollution is sometimes referred to as "black carbon" pollution.⑤The exhaust from burning fuels in automobiles, homes, and industries is a major source of pollution in the air.⑥Some authorities believe that even the burning of wood and charcoal in fireplaces and barbeques can release significant quantities of soot into the air.一类空气污染是在燃烧燃料获取能源时将粒子排放到空气中。
土壤碳氮迁移水动力学机制
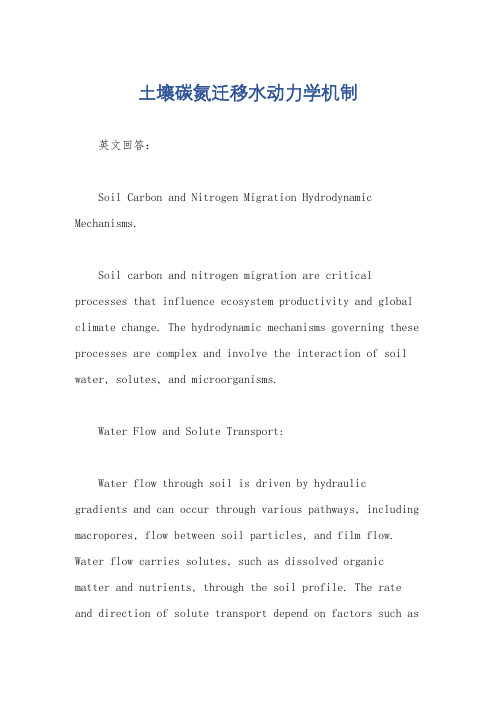
土壤碳氮迁移水动力学机制英文回答:Soil Carbon and Nitrogen Migration Hydrodynamic Mechanisms.Soil carbon and nitrogen migration are critical processes that influence ecosystem productivity and global climate change. The hydrodynamic mechanisms governing these processes are complex and involve the interaction of soil water, solutes, and microorganisms.Water Flow and Solute Transport:Water flow through soil is driven by hydraulic gradients and can occur through various pathways, including macropores, flow between soil particles, and film flow. Water flow carries solutes, such as dissolved organic matter and nutrients, through the soil profile. The rate and direction of solute transport depend on factors such asthe soil's hydraulic conductivity, porosity, and solute concentration.Hydrological Connectivity:Hydrological connectivity refers to the degree of connection between different parts of the soil system. It influences the movement and retention of water and solutes. Well-connected soils allow for the rapid transport of solutes over long distances, while poorly connected soils limit solute movement.Soil Structure and Pore Space:Soil structure and pore space play a crucial role in carbon and nitrogen migration. Macropores facilitate rapid water and solute movement, while micropores promote solute retention and microbial activity. Soil compaction and other disturbances can alter the soil structure, affecting the hydrodynamic mechanisms and solute transport.Microbial Activity and Biogeochemical Processes:Microorganisms play a vital role in the decomposition and mineralization of soil organic matter, which release carbon and nitrogen into the soil solution. Microbial activity is influenced by soil moisture, temperature, and nutrient availability. Biogeochemical processes, such as nitrification and denitrification, also contribute to the transformation and migration of nitrogen in the soil.Hydrological Extremes and Solute Leaching:Hydrological extremes, such as droughts and floods, can have significant impacts on carbon and nitrogen migration. Droughts reduce water flow and solute transport, leading to the accumulation of solutes in the soil profile. Floods, on the other hand, can rapidly transport solutes through the soil, leading to leaching losses.Management Implications:Understanding the hydrodynamic mechanisms governing carbon and nitrogen migration is crucial for developingeffective soil management practices. By controlling water flow, managing soil structure, and optimizing microbial activity, it is possible to enhance soil carbon and nitrogen retention, reduce nutrient losses, and mitigate the impacts of hydrological extremes.中文回答:土壤碳氮迁移水动力学机制。
油气净化大师
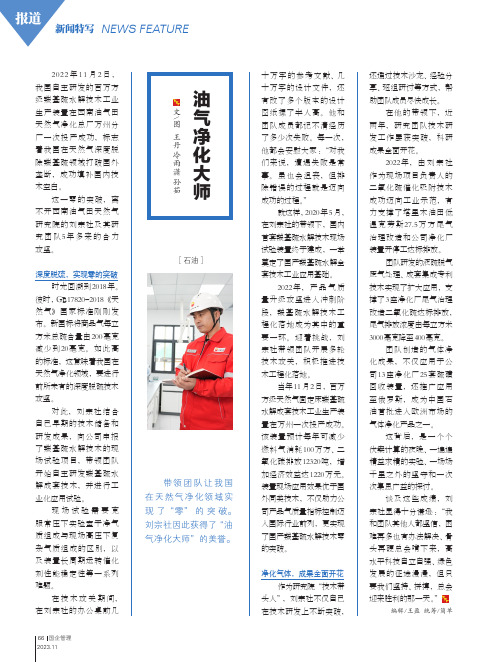
[石油]2022年11月2日,我国自主研发的百万方级羰基硫水解技术工业生产装置在西南油气田天然气净化总厂万州分厂一次投产成功,标志着我国在天然气深度脱除羰基硫领域打破国外垄断,成功填补国内技术空白。
这一零的突破,离不开西南油气田天然气研究院的刘宗社及其研究团队5年多来的合力攻坚。
深度脱硫,实现零的突破时光回溯到2018年。
彼时,GB 17820-2018《天然气》国家标准刚刚发布。
新国标将商品气每立方米总硫含量由200毫克减少到20毫克。
如此高的标准,这意味着我国在天然气净化领域,要进行前所未有的深度脱硫技术攻坚。
对此,刘宗社结合自己早期的技术储备和研发成果,向公司申报了羰基硫水解技术的现场试验项目,带领团队开始自主研发羰基硫水解成套技术,并进行工业化应用试验。
现场试验需要克服常压下实验室干净气质组成与现场高压下复杂气质组成的区别,以及装置长周期运转催化剂性能稳定性等一系列难题。
在技术攻关期间,在刘宗社的办公桌前几文\图 王丹 冷雨潇孙茹十万字的参考文献、几十万字的设计文件,还有改了多个版本的设计图纸摞了半人高。
他和团队成员都记不清经历了多少次失败。
每一次,他都会安慰大家:“对我们来说,遭遇失败是常事。
虽也会沮丧,但排除错误的过程就是迈向成功的过程。
”就这样,2020年5月,在刘宗社的带领下,国内首套羰基硫水解技术现场试验装置终于建成,一举奠定了国产羰基硫水解全套技术工业应用基础。
2022年,产品气质量升级攻坚进入冲刺阶段,羰基硫水解技术工程化落地成为其中的重要一环。
迎着挑战,刘宗社带领团队开展多轮技术攻关,积极推进技术工程化落地。
当年11月2日,百万方级天然气固定床羰基硫水解成套技术工业生产装置在万州一次投产成功。
该装置预计每年可减少燃料气消耗100万方、二氧化碳排放12320吨,增加经济效益达1220万元。
装置现场应用效果优于国外同类技术,不仅助力公司产品气质量指标控制迈入国际行业前列,更实现了国产羰基硫水解技术零的突破。
惰气熔融-红外吸收
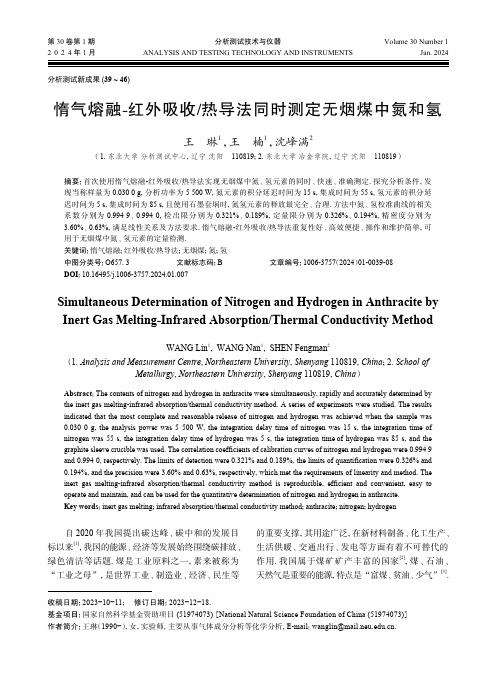
分析测试新成果 (39 ~ 46)惰气熔融-红外吸收/热导法同时测定无烟煤中氮和氢王 琳1,王 楠1,沈峰满2(1. 东北大学 分析测试中心,辽宁 沈阳 110819;2. 东北大学 冶金学院,辽宁 沈阳 110819)摘要:首次使用惰气熔融-红外吸收/热导法实现无烟煤中氮、氢元素的同时、快速、准确测定. 探究分析条件,发现当称样量为0.030 0 g ,分析功率为5 500 W ,氮元素的积分延迟时间为15 s ,集成时间为55 s ,氢元素的积分延迟时间为5 s ,集成时间为85 s ,且使用石墨套埚时,氮氢元素的释放最完全、合理. 方法中氮、氢校准曲线的相关系数分别为0.994 9、0.994 0,检出限分别为0.321%、0.189%,定量限分别为0.326%、0.194%,精密度分别为3.60%、0.63%,满足线性关系及方法要求. 惰气熔融-红外吸收/热导法重复性好、高效便捷、操作和维护简单,可用于无烟煤中氮、氢元素的定量检测.关键词:惰气熔融;红外吸收/热导法;无烟煤;氮;氢中图分类号:O657. 3 文献标志码:B 文章编号:1006-3757(2024)01-0039-08DOI :10.16495/j.1006-3757.2024.01.007Simultaneous Determination of Nitrogen and Hydrogen in Anthracite by Inert Gas Melting-Infrared Absorption/Thermal Conductivity MethodWANG Lin 1, WANG Nan 1, SHEN Fengman2(1. Analysis and Measurement Centre , Northeastern University , Shenyang 110819, China ;2. School ofMetallurgy , Northeastern University , Shenyang 110819, China )Abstract :The contents of nitrogen and hydrogen in anthracite were simultaneously, rapidly and accurately determined by the inert gas melting-infrared absorption/thermal conductivity method. A series of experiments were studied. The results indicated that the most complete and reasonable release of nitrogen and hydrogen was achieved when the sample was 0.030 0 g, the analysis power was 5 500 W, the integration delay time of nitrogen was 15 s, the integration time of nitrogen was 55 s, the integration delay time of hydrogen was 5 s, the integration time of hydrogen was 85 s, and the graphite sleeve crucible was used. The correlation coefficients of calibration curves of nitrogen and hydrogen were 0.994 9and 0.994 0, respectively. The limits of detection were 0.321% and 0.189%, the limits of quantification were 0.326% and 0.194%, and the precision were 3.60% and 0.63%, respectively, which met the requirements of linearity and method. The inert gas melting-infrared absorption/thermal conductivity method is reproducible, efficient and convenient, easy to operate and maintain, and can be used for the quantitative determination of nitrogen and hydrogen in anthracite.Key words :inert gas melting ;infrared absorption/thermal conductivity method ;anthracite ;nitrogen ;hydrogen自2020年我国提出碳达峰、碳中和的发展目标以来[1],我国的能源、经济等发展始终围绕碳排放、绿色清洁等话题. 煤是工业原料之一,素来被称为“工业之母”,是世界工业、制造业、经济、民生等的重要支撑,其用途广泛,在新材料制备、化工生产、生活供暖、交通出行、发电等方面有着不可替代的作用. 我国属于煤矿矿产丰富的国家[2],煤、石油、天然气是重要的能源,特点是“富煤、贫油、少气”[3].收稿日期:2023−10−11; 修订日期:2023−12−18.基金项目:国家自然科学基金资助项目 (51974073) [National Natural Science Foundation of China (51974073)]作者简介:王琳(1990−),女,实验师,主要从事气体成分分析等化学分析,E-mail :****************.第 30 卷第 1 期分析测试技术与仪器Volume 30 Number 12024年1月ANALYSIS AND TESTING TECHNOLOGY AND INSTRUMENTS Jan. 2024煤根据品种及品质的不同,分为烟煤、无烟煤、焦炭等,并应用于不同行业,其中无烟煤因其燃烧无烟、煤化程度高、含碳量高、热值高、挥发分低等特点,普遍用于燃料及燃料电池、先进碳材料[4-7]、催化剂[8]、吸附剂[9-10]、滤料、民用煤等. 而据统计显示,我国空气污染源中的粉尘、PM2.5、SO2及NO x等大部分来自于民用煤燃烧的排放[11],因此加强对无烟煤的质量监测,是提升煤炭质量、发展低碳与绿色能源的重要环节.煤炭的检测标准溯源到上世纪60年代,检测指标一般包括工业分析[12](水分、灰分、挥发分、固定碳)、元素分析[13-15](C、S、O、N、H)、有价元素分析[16-17](As、Ga、Se、Ge等)、阴离子[18](氟等)等. 其中无烟煤中的氮元素在燃烧后会形成NO x,对人类及居住环境污染影响较大[11]. 无烟煤中氢元素含量的多少,代表了热值的大小. 因此准确快速测定无烟煤中氮、氢含量对煤炭质量控制,煤炭行业的检验检测、标准制定、能源开发及环境保护等均具有重要意义.对于无烟煤中氮、氢元素的检测,通常使用半微量开氏法和半微量蒸汽法[19]、高温燃烧-检测器测定法[14, 20]测定无烟煤中的氮含量,采用三节炉法、二节炉法[13]、电量-重量法[21]、高温燃烧-检测器测定法[14]测定无烟煤中的氢含量. 其中三节炉法、二节炉法、电量-重量法均存在硫、氯等元素的干扰,需使用铬酸铅、银丝、二氧化锰等试剂消除干扰,污染较大且成本高. 随着科技的进步,仪器法逐渐被用于测定无烟煤中的氮、氢元素含量,现有的仪器法[22]原理是将无烟煤在氧气下燃烧,对燃烧生成的H2O、N2气体进行检测. 但该法存在燃烧炉/管升降温时间长、分析时间长、维护复杂、耗材昂贵等缺点. 而以惰气熔融-红外吸收/热导法为分析原理设计的氧氮氢分析仪通常用于陶瓷、粉末[23]、钢铁[24]等无机材料中氧、氮、氢元素的测定,并以快速、精准的优势成为冶金、材料等领域以及检验检测机构在气体元素分析方面的常用仪器. 但目前为止,未见其应用于无烟煤类产品的检测工作中,其在使用中无需强酸、重金属等试剂,具有无需等待升降温、分析时间短、样品前处理简易、维护相对简单等优势,满足绿色、安全、快速、准确分析的要求,因此本文首次尝试将惰气熔融-红外吸收/热导法应用于无烟煤中氮、氢元素的检测.1 试验部分1.1 仪器与试剂氧氮氢分析仪:美国力可公司,ONH836;天平:赛多利斯,SQP;石墨套埚(内坩埚加外坩埚)、石墨标准坩埚、镍嚢,LECO公司;有机元素分析仪:德国元素公司,Vario MACRO cube.氦气(99.999%),氮气(99.5%),沈阳顺泰特种气体有限公司;无烟煤标准物质:ZBM093、ZBW112A、ZBM095A,济南众标科技有限公司生产;GBW11104j,国家煤炭质量监督检验中心;GBW11108o,山东省冶金科学研究院. 对氨基苯磺酰胺(C6H8N2O2S)、WO3,德国元素公司;未知样品为某学生客户日常送检的无烟煤样品.1.2 试验原理在惰性气体氦气保护下,样品置于上下电极间的石墨坩埚中,经过坩埚脱气、吹扫、脉冲炉通电,上、下电极及石墨坩埚形成电路并加热,使待测样品完全熔融,N、H元素分别以N2、H2分子形式释放,随载气氦气流经热的氧化铜催化剂,H2被完全氧化成H2O,N2、H2O一起进入红外检测池,根据H2O的特征红外吸收波长,检测得到氢元素的含量,之后H2O被高氯酸镁等过滤试剂吸收,N2进入热导检测池完成氮元素的测定,其原理图如图1所示.样品上电级红外检测池检测 H2O热导检测池检测 N2坩埚下电极脉冲熔融炉N2N2H2催化剂H2OH2O图1 氧氮氢分析仪测定氮、氢的工作原理图Fig. 1 Working principle diagram ofOxygen/Nitrogen/Hydrogen Analyzer determined nitrogenand hydrogen1.3 试验方法1.3.1 准备工作将标准物质、待测样品置于110 ℃洁净的烘箱中烘干2 h,保证粒度在0.074 mm以下,然后再置40分析测试技术与仪器第 30 卷于干燥器中冷却备用.对氧氮氢分析仪进行彻底维护,包括上电极、下电极、投样口的清扫清洁,催化剂、过滤试剂等试剂的更换,并通过漏气检查,保证仪器的气密性.1.3.2 试验步骤打开稳压电源、氧氮氢分析仪主机及软件,将下电极升高,在氦气保护模式下进行仪器预热至少1 h,预热完成后打开氦气至流速为450 mL/min,开通冷却水,使检测器保持在稳定的工作温度. 本方法以镍嚢及空白石墨套锅作为空白,分别称取0.010 0~0.100 0 g(精确到±0.000 3 g)的样品,小心倾倒于镍嚢内,等待投样,设置4 500~6 000 W的分析功率,对比石墨套埚与石墨标准坩埚的分析效果,分别设置0~15 s的分析延迟时间、50~85 s数据集成时间等仪器参数. 开始测试后进行投放样品、取下坩埚、更换新的内坩埚、脱气、吹扫等操作,依次进行空白、标准物质及未知样品的测试,建立标准曲线,并对方法进行检出限、定量限、精密度等试验验证.1.3.3 未知样品对比试验本文使用有机元素分析仪作为未知样品测试的对比方法,并命名为方法1. 对有机元素分析仪(CHNS模式)的燃烧管进行清理并更换试剂及灰分坩埚,还原管内铜及银丝重新装填,酒精擦拭干净后放回到炉子内,通高纯氦气,流速为600 mL/min,室温检漏通过后,分别升至1 150、850 ℃工作温度下吹扫4 h后进行试验. 使用仪器自带标准曲线,以75 mg的锡纸包裹,称取25 mg的对氨基苯磺酰胺作为“run”和漂移标准物质进行曲线校正,待测样品称样量为50 mg,加入WO3助熔,75 mg锡纸包裹,使用工具压除空气后置于自动进样器中进样,试样在1 150 ℃下通高纯氧气燃烧,850 ℃下催化还原,释放出N2和H2O,进入相应检测池分析检测,经过“吹扫-捕集”吸附解析的分离过程,得到氮、氢的分析数据,完成检测.2 结果与讨论2.1 进样方式的确定本试验采用直投法进样,对于粉末类样品以此方式进样时,会造成进样系统污染、进样量减少、分析数据偏低等问题,为避免因进样造成的分析误差,需采用镍嚢作为样品包裹体,保证进样量的准确性及释放完全性.2.2 进样量的确定样品的进样量会影响熔融效果,使用标准物质ZBM095A作为待测样品,对比0.010 0、0.020 0、0.030 0、0.040 0、0.050 0、0.060 0、0.080 0、0.100 0 g 进样量对氮、氢元素释放效果的影响. 由图2可见,随着进样量的增加,氮质量比在进样量为0.010 0~ 0.030 0 g时的测定结果变化不大,而在0.0300 g时出现拐点呈下降趋势,随着进样量的继续增加,由于释放条件不足,氮质量比下降,因此氮的最佳进样量为0.0300 g. 氢质量比随进样量增加,先呈明显上升趋势,在进样量为0.030 0 g时,氢质量比达到了最高点,而随着进样量的继续增大,氢质量比缓慢降低,在进样量大于0.060 0 g时,氢质量比迅速下降. 由此可见,0.0300 g是其最佳进样量. 产生该现象的原因可能是进样量较低时,样品分析浓度不够,导致氢元素质量比偏低,而进样量过高时,样品的分析条件不足以使氢完全释放,氢元素质量比降低,且就仪器本身的检测范围而言,氢的测量上限绝对质量为0.002 5 g,因此对于标准物质ZBM095A 的氢元素质量比的测定,当进样量超过0.050 0 g时,检测池处于饱和状态,无法正常检测. 因此,0.030 0 g 为该方法的最佳进样质量.4.54.03.53.02.52.01.51.00.500.020 00.040 00.060 0NH0.080 00.100 0m/g质量比/%图2 不同进样量下氮、氢的测试结果Fig. 2 Test results of nitrogen and hydrogen underdifferent sample masses2.3 分析功率的确定在氮、氢元素分析中,分析功率是决定样品释放的重要参数. 本试验依次设置4 500、5 000、5 500、6 000 W的功率梯度,观察功率对于无烟煤中氮、氢元素检测的影响. 图3为氮、氢的测试值随功率变化的关系图. 由图3可见,当功率较低,在4 500、5 000 W时,氮、氢元素质量比偏低,说明过低的功第 1 期王琳,等:惰气熔融-红外吸收/热导法同时测定无烟煤中氮和氢41率不足以使无烟煤完全熔融释放,这与无烟煤本身含碳量高、燃点高的特性一致. 但当功率为6 000 W 时,质量比再次下降,这是因为功率过高,导致氮、氢元素过早溢出,数据捕捉不及时,导致数据偏低.当分析功率为5 500 W 时,氮、氢元素的释放最完全,测定值最高. 由此可见,无烟煤的最佳分析功率为5 500 W.2.4 分析坩埚的对比氮、氢元素分析的样品载体一般分为石墨套埚(外坩埚加内坩埚)和标准坩埚. 本试验对比二者的分析效果,观察图4(a )的氮元素及图4(b )的氢元素在使用不同坩埚时的测定谱图,可发现氮、氢元素在使用石墨套埚得到的测定值明显高于标准坩埚,说明石墨套埚的分析效果优于标准坩埚. 究其原因,标准坩埚对比石墨套埚来说相对单薄,在5 500 W 的高功率下其承压能力小,甚至存在标准坩埚被烧漏或者断裂的情况,因而标准坩埚的使用会导致数据偏低,对于无烟煤这类燃点高、熔融产生热量大的样品来说,双层结构的套埚更适用. 因此,本试验选用石墨套埚作为分析坩埚.2.5 分析参数的设定(包括分析延迟时间、数据集成时间)本方法对仪器分析参数(分析延迟时间、数据集成时间)进行了探究. 对比了15、10、5、0 s 四种延迟时间,观察图5(a )可见,15 、10 s 时氢的出峰过早、不完整且峰形不佳,导致氢元素的数据捕集不完全,测试数据偏低. 当调整为5 s 时,氢峰的前端有平缓的基线,0 s 时出峰过缓. 因此,5 s 是合理的延迟时间. 由图5(b )可见,氮的测试值随延迟时间的增加而增大,其延迟时间设置为15 s 较合理.对于出峰不完全的问题,本试验采用将数据集成时间延长的方式,分别设置为55、65、75、80、85 s ,观察图6(a )发现,当集成时间为55、65、75 s 时,氢峰的末端均未回到基线的位置,数据偏低. 80 s 时谱线回到基线,85 s 时形成相对完整的正态分布峰,与图6(b )的数据趋势吻合. 同时观察图6(b )发现,氮的集成时间为55s 数据更合理. 因此本方法选择氮的延迟时间为15 s 、集成时间为55 s ,氢的延迟时间为5 s 、集成时间为85 s 为最佳分析参数.2.6 标准曲线建立及检出限测定无烟煤中的氮、氢元素含量范围较宽泛,单点校准的方式并不适用. 本文采用建立标准曲线的校准方式,在称样质量为0.030 0 g 、分析功率为5 500W ,氮、氢元素延迟时间分别为15、5 s ,捕集时间分别为55、85 s ,使用石墨套埚的试验条件下,选择有证标准物质ZBM093、GBW11104j 、GBW11108o 、2.754.34.24.14.03.92.702.652.602.554 5005 000N H5 5006 000P /W质量比/%质量比/%图3 分析功率的探究试验Fig. 3 Test results of nitrogen and hydrogen underdifferent analytical powers100(a)608040积分强度石墨套锅标准坩埚2000102030t /s405060100(b)608040积分强度石墨坩埚标准坩埚2000102030t /s405060图4 石墨套埚与标准坩埚的确定试验(a)不同坩埚对氮元素的测试谱图,(b)不同坩埚对氢元素的测试谱图Fig. 4 Comparison of test results between graphite sleeve pote and standard crucible (a) spectra of nitrogen in different crucibles, (b) spectra of hydrogen in different crucibles42分析测试技术与仪器第 30 卷ZBW112A 建立标准曲线,其认定值及测量值结果如表1所列. 氮、氢元素的线性方程分别为:Y =2.098 404 22X −0.000 200 66、Y =0.789 376 46X −0.000 044 57,相关系数分别为0.994 9、0.994 0,满足线性关系. 对空白坩埚连续测试11次,得到氮、氢元素的平均值分别为0.318 9%、0.186 9%,以该结果与3倍标准偏差之和作为检出限,分别为0.321%、0.189%,以平均值与10倍标准偏差之和作为定量限,分别为0.326%、0.194%,结果如表2所列,表明该方法检测范围较宽,适用于无烟煤中氮、氢元素的定量检测.2.7 方法的准确度、精密度测试精密度测试是验证方法可靠性的重要指标,本试验使用有证无烟煤标准物质ZBM095A 进行精密度测试,平行测定7次,并计算其精密度. 如表3所列,其氮、氢元素的测定平均值分别为1.30%、3.30%,由表1可知,其认证值分别为1.31%±0.07%、3.23%±0.10%,因此该方法准确度较好. 经计算,氮、氢的精密度分别为3.60%、0.63%,满足方法精密度要求. 由此可见该方法准确可靠.表 1 标准物质及其认证值、测量值Table 1 Certified and measured values of standardsubstances/%标准物质NH 认证值测量值认证值测量值ZBM0930.56±0.060.563 3.01±0.12 2.92GBW11104j 0.94±0.070.929 2.64±0.15 2.71GBW11108o 1.30±0.06 1.30 4.58±0.13 4.59ZBW112A 1.10±0.06 1.12 3.78±0.10 3.79ZBM095A1.31±0.071.303.23±0.103.3010015 s 10 s 5 s 0 s(a)8060积分强度402005101520253035t /s 404550556065702.655.04.03.02.01.00N H(b)2.602.552.50质量比/%质量比/%2.452.402.3551015t /s图5 氮、氢的分析延迟时间对比试验(a) 不同延迟时间下氢的测试谱图, (b)延迟时间对氮、氢的影响Fig. 5 Comparison test of analysis delay times of nitrogen and hydrogen(a) spectra of hydrogen in different delay times, (b) effect of delay times on nitrogen and hydrogen100 2.705.04.94.84.74.62.682.662.642.622.6055606570758085909585 s 80 s 75 s 65 s 55 s806040积分强度质量比/%质量比/%20002040t /st /s6080100(a)(b)图6 氮、氢的集成时间对比试验(a)不同集成时间下氢的测试谱图, (b)集成时间对氮、氢的影响Fig. 6 Comparison test of integration times of nitrogen and hydrogen(a) spectra of hydrogen in different integration times, (b) effect of integration times on nitrogen and hydrogen第 1 期王琳,等:惰气熔融-红外吸收/热导法同时测定无烟煤中氮和氢432.8 未知样品测试对日常送检的无烟煤样品进行抽检,并标号为样品1、样品2,使用方法1与本方法进行对比,随试验进行ZBM095A的测试. 分别平行测定7次,其测试结果如表4所列. 由表可见,方法1测得样品1、样品2、ZBM095A中氮的平均值分别为0.096 6%、1.086%、1.30%,相对标准偏差(RSD)分别为2.67%、1.75%、3.60%. 氢的平均值分别为2.899%、3.312%、3.30%,RSD分别为1.90%、1.50%、0.63%. 本方法测得样品1、样品2、ZBM095A中氮的平均值分别为0.094 6%、1.067%、1.25%,RSD分别为2.99%、1.69%、3.90%. 氢的平均值分别为2.927%、3.300%、3.20%,RSD分别为1.87%、1.56%、0.72%. 对比两种方法,准确度与精密度均能够满足试验要求,再次证实本文建立的方法适用于无烟煤中的氮、氢两种元素的定量测定.表 3 ZBM095A的精密度试验Table 3 Precision test of ZBM095A/%元素测定值平均值RSDN 1.28、1.26、1.34、1.35、1.36、1.30、1.24 1.30 3.60H 3.30、3.32、3.33、3.29、3.29、3.33、3.28 3.300.63表 4 两种方法测试未知样品的对比试验Table 4 Comparison of two methods for testing unknown samples/%样品方法1平均值方法1 RSD本方法平均值本方法RSD N H N H N H N H样品10.096 6 2.899 2.67 1.900.094 6 2.927 2.99 1.87样品 2 1.086 3.312 1.75 1.50 1.067 3.300 1.69 1.56 ZBM095A 1.30 3.30 3.600.63 1.25 3.20 3.900.723 结论(1)本文首次将惰性气体熔融-红外吸收/热导法应用于无烟煤类产品的检测中,该方法满足同时、快速、准确的特点,减少了强酸化学试剂的使用,体现了绿色化学宗旨.(2)建立了无烟煤中氮、氢元素定量测试的方法,为煤炭行业的检验检测、标准制定、贸易等提供参考.(3)拓展了氧氮氢分析仪的使用范围,在有色金属、高温合金、难熔金属、稀土、陶瓷、矿石等材料的使用范围之外,增加了无烟煤类产品的使用.参考文献:习近平. 在第七十五届联合国大会一般性辩论上的讲话[N]. 人民日报, 2020-09-23(3).[ 1 ]元雪芳, 任恒星, 郭鑫, 等. 不同物质对无烟煤生物转化的影响研究[J].煤化工,2022,50(5):79-82.[YUAN Xuefang, REN Hengxing, GUO Xin, et al.Study on impact of adding different substances on bio-transformation of anthracite[J]. Coal Chemical In-dustry,2022,50 (5):79-82.][ 2 ]吕俊复, 蒋苓, 柯希玮, 等. 碳中和背景下循环流化床燃烧技术在中国的发展前景[J]. 煤炭科学技术,2023,51(1):514-522. [LV Junfu, JIANG Ling, KEXiwei, et al. Future of circulating fluidized bed com-[ 3 ]表 2 方法的线性与检出限Table 2 Linearity and limits of detection元素线性方程线性相关系数(R2)平均值/(%,n=11)检出限/%定量限/% N Y=2.098 404 22X − 0.000 200 660.994 90.318 90.3210.326H Y=0.789 376 46X − 0.000 044 570.994 00.186 90.1890.19444分析测试技术与仪器第 30 卷bustion technology in China for carbon neutralization [J ]. Coal Science and Technology ,2023,51 (1):514-522.]于昭仪, 谢卫宁, 邱钿, 等. 添加剂对煤基石墨微观结构的影响[J ]. 煤炭科学技术,2023,51(5):302-308.[YU Zhaoyi, XIE Weining, QIU Tian, et al. Effect of additives on microstructure of coal-based graphite [J ].Coal Science and Technology ,2023,51 (5):302-308.][ 4 ]传秀云, 鲍莹. 煤制备新型先进炭材料的应用研究[J ]. 煤炭学报,2013,38(S1):187-194. [CHUAN Xiuyun, BAO Ying. Application of coal as raw materi-als in preparing new advanced carbon materials [J ].Journal of China Coal Society ,2013,38 (S1):187-194.][ 5 ]唐跃刚, 陈鹏翔, 李瑞青, 等. 京西煤制备氧化石墨烯分子结构模型的构建与优化[J ]. 煤炭科学技术,2021,49(6):126-134. [TANG Yuegang, CHEN Pengxiang, LI Ruiqing, et al. Model construction and optimization of molecule structure of coal-based grapheme oxide from Jingxi coal [J ]. Coal Science and Technology ,2021,49 (6):126-134.][ 6 ]Zhang C, Xie Y C, Zhang C, et al. Upgrading coal tomultifunctional graphene-based materials by direct laser scribing [J ]. Carbon ,2019,153 :585-591.[ 7 ]杨丽, 刘帅, 辛春梅, 等. 炭黑负载增加活性炭缺陷位点催化甲烷裂解制氢[J/OL ]. 煤炭科学技术, 2023:1-11[2023-12-08]. https:///kcms/detail/11.2402.td.20230706.1933.007.html. [YANG Li, LIU Shuai, XIN Chunmei, et al. The loading of carbon black increased the defect site of activated carbon Catalytic pyrolysis of methane for hydrogen produc-tion [J/OL ]. Coal science and technology, 2023: 1-11[2023-12-08]. https:///kcms/detail/11.2402.td.20230706.1933.007.html.][ 8 ]张昆, 孟召平, 金毅, 等. 不同煤体结构煤的孔隙结构分形特征及其研究意义[J ]. 煤炭科学技术,2023,51(10):198-206. [ZHANG Kun, MENG Zhaoping,JIN Yi, et al. Fractal characteristics of pore structures on different coal structures and its research signific-ance [J ]. Coal Science and Technology ,2023,51 (10):198-206.][ 9 ]狄军贞, 曹洋, 赵文琦. 巯基改性褐煤的制备及其对Fe 2+、Mn 2+的吸附特性研究[J ]. 煤炭科学技术,2023,51(3):261-270. [DI Junzhen, CAO Yang, ZHAO Wenqi. Preparation of mercapto modified lignite and its adsorption characteristics for Fe 2+、Mn 2+[J ]. Coal[ 10 ]Science and Technology ,2023,51 (3):261-270.]何绪文. 民用燃煤大气污染物控排技术对策[J ]. 洁净煤技术,2017,23(4):12-17. [HE Xuwen. Counter-measure of air pollutant controlled pollutation for ci-vilian coal [J ]. Clean Coal Technology ,2017,23 (4):12-17.][ 11 ]国家质量监督检验检疫总局, 中国国家标准化管理委员会. 煤的工业分析方法 仪器法: GB/T 30732—2014[S ]. 北京: 中国标准出版社, 2014. [General Administration of Quality Supervision, Inspection and Quarantine of the People's Republic of China,Standardization Administration of the People's Re-public of China. Proximate analysis of coal-instru-mental method: GB/T 30732—2014[S ]. Beijing:Standards Press of China, 2014.][ 12 ]国家质量监督检验检疫总局. 煤的元素分析方法:GB/T 476—2001[S ]. 北京: 中国标准出版社, 2001.[General Administration of Quality Supervision, In-spection and Quarantine of the People's Republic of China. Ultimate analysis of coal: GB/T476—2001[S ]. Beijing: Standards Press of China,2001.][ 13 ]国家质量监督检验检疫总局, 中国国家标准化管理委员会. 煤中碳氢氮的测定 仪器法: GB/T 30733—2014[S ]. 北京: 中国标准出版社, 2014. [General Administration of Quality Supervision, Inspection and Quarantine of the People's Republic of China,Standardization Administration of the People's Re-public of China. Determination of total carbon, hy-drogen and nitrogen content in coal-instrumental method: GB/T 30733—2014[S ]. Beijing: Standards Press of China, 2014.][ 14 ]杜强, 王喜武. 煤直接液化工艺硫氮元素分布及其影响研究[J ]. 中国煤炭,2022,48(8):104-108. [DU Qiang, WANG Xiwu. Research on the distribution of sulfur and nitrogen and its influence in direct coal li-quefaction process [J ]. China Coal ,2022,48 (8):104-108.][ 15 ]国家市场监督管理总局, 国家标准化管理委员会.煤中有价元素含量分级及应用导则: GB/T 41042—2021[S ]. 北京: 中国标准出版社, 2021.[State Administration for Market Regulation, Na-tional Standardization Administration. Guidance for utilization and classification of content of valuable elements in coal: GB/T 41042—2021[S ]. Beijing:Standards Press of China, 2021.][ 16 ]姬晓燕, 张志峰, 祁风华, 等. 任家庄井田晚古生代煤[ 17 ]第 1 期王琳,等:惰气熔融-红外吸收/热导法同时测定无烟煤中氮和氢45系战略性金属元素富集特征[J/OL ]. 煤炭科学技,2023: 1-15 [2023-12-08]. https://doi. org/10.13199/ki.cst.2023-0146. [JI Xiaoyan, ZHANG Zhifeng, QI Fenghua, et al. Characteristics of strategic metal ele-ment enrichment in late Paleozoic coal measures in Renjiazhuang Jingtian [J/OL ]. Coal Science and Technology, 2023: 1-15[2023-12-08]. https:///10.13199/ki.cst.2023-0146.]唐佳伟, 张锁, 刘兆峰, 等. 吸附法去除水中F -进展及其在矿井水处理中发展方向[J/OL ]. 煤炭科学技术,2023: 1-16[2023-12-08]. https: //doi. org/10.13199/ki. cst. 2022-1835. [TANG Jiawei, ZHANG Suo,LIU Zhaofeng, et al. Progress in removal of F -in wa-ter by adsorption method and its development direc-tion in mine water treatment [J/OL ]. Coal science and technology, 2023: 1-16[2023-12-08]. https:///10.13199/ki.cst.2022-1835.][ 18 ]国家质量监督检验检疫总局, 中国国家标准化管理委员会. 煤中氮的测定方法: GB/T 19227—2008[S ]. 北京: 中国标准出版社, 2009. [General Administration of Quality Supervision, Inspection and Quarantine of the People's Republic of China,Standardization Administration of the People's Re-public of China. Determination of nitrogen in coal:GB/T 19227—2008[S ]. Beijing: Standards Press of China, 2009.][ 19 ]龚婉莉. 采用元素分析仪测定煤中碳氢氮含量的应用研究[J ]. 煤质技术,2018(1):38-41, 49. [GONG Wanli. The application study on determination of car-bon, hydrogen and nitrogen content in coal by ele-mental analyzer [J ]. Goal Quality Technology ,2018(1):38-41, 49.][ 20 ]国家质量监督检验检疫总局, 中国国家标准化管理委员会. 煤中碳和氢的测定方法: GB/T 476—2008[S ]. 北京: 中国标准出版社, 2009. [General Administration of Quality Supervision, Inspection and Quarantine of the People's Republic of China,Standardization Administration of the People's Re-public of China. Determination of carbon and hydro-gen in coal: GB/T 476—2008[S ]. Beijing: Standards Press of China, 2009.][ 21 ]孙玉芳, 王曦. 元素分析仪测定土壤氮、碳含量的不确定度评定[J ]. 分析测试技术与仪器, 2016, 22(4):240-245. [SUN Yufang, WANG Xi. Uncertainty eval-uation of measurement results for determination of total carbon and nitrogen in soil samples using ele-mental analyzer [J ]. Analysis and testing technology and instruments. 2016, 22(4): 240-245.][ 22 ]朱春要, 秦建, 赵希文, 等. 脉冲熔融-红外/热导法测定钛合金粉末微注射成形脱脂坯中氧氮氢[J ]. 中国无机分析化学,2023,13(5):499-504. [ZHU Chunyao, QIN Jian, ZHAO Xiwen, et al. Determina-tion of oxygen, nitrogen and hydrogen in micro injec-tion molding of titanium alloy powder about degrease billets by impulse fusion-infrared absorption and thermal conductivity method [J ]. Chinese Jorunal of Inorganic Analytical Chemistry ,2023,13 (5):499-504.][ 23 ]侯桂臣, 张琳, 李一懿, 等. “直投法” 应用于金属粉末样品中氧氮氢分析[J ]. 冶金分析,2019,39(6):7-13. [HOU Guichen, ZHANG Lin, LI Yiyi, et al. Ap-plication of "direct-dropping" for analysis of oxygen,nitrogen and hydrogen in metal powder sample [J ].Metallurgical Analysis ,2019,39 (6):7-13.][ 24 ]46分析测试技术与仪器第 30 卷。
碳约束氮化铁纳米材料制备成功
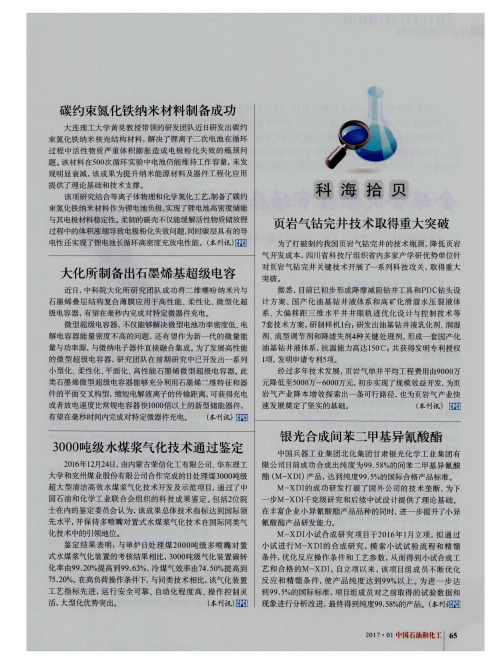
岩气 产业 降本增效探索出一条可行路径, 也为页岩气产业快
速 发展 奠 定了坚实 的基 础。 ( 表 刊讯) 田 珂
或者放 电速 度 比常规 电容器 快1 0 0 0 倍 以上 的新型 储能器 件, 有 望在毫 秒时 间内完成对 特定微器 件充电。 ( 本刊讯)r 8 珂
的微 型 超级 电容 器 , 研 究 团 队在 前 期研 究 中已开发 出一系列
剂、 流 型调 节剂 和降滤 失剂4 种 关键 处理 剂, 形成一 套 国产 化 油 基 钻井 液体 系, 抗 温能力 高达 1 5 0  ̄ C ; 共 获得发 明专利授 权 1 项, 发明 申 请 专 利5 项。
3 0 0 0 吨 级 水煤 浆气化 技 术通 过 鉴定
2 0 1 6 年l 2 月2 4 日, 由内蒙古荣 信化 工有 限公司、 华东理 工
银光 合成 间苯二 甲基 异 氰酸 酯
中国 兵器 工 业 集 团北 化 集 团甘 肃 银 光化 学 工业 集 团有 限 公司 日前成 功 合 成 出纯 度 为9 9 . 5 8 %的 间苯 二 甲基 异 氰 酸
鉴 定结 果 表 明 : 与 置 化 率 由9 9 . 2 0 % 提高 ̄ 1 1 9 9 . 6 3 %, 冷 煤 气 效率 由7 4 . 5 0 %提 高到
式水煤 浆气化 装置 的考 核结果 相 比, 3 0 0 0 吨级 气化 装置 碳转 条 件 , 优化 反 应操 作 条 件和 工艺 参 数 , 从而 得 到小试 合成 工 艺和 合格 的M— XDI 。 自立 项 以 来 , 该 项 目组 成 员不断 优化 7 5 . 2 O %。 在高负荷操作条件下, 与同类技术相比, 该气化装置 反 应 和 精 馏 条 件 , 使 产 品纯 度 达 到 9 9 %以上 。 为 进 一 步 达 工艺 指 标 先进 , 运行 安 全 可靠 、 自 动化程度高、 操 作 控 制灵  ̄ 1 1 9 9 . 5 %的 国际标 准 , 项目 组 成 员对 之前取 得 的试 验 数据 和 活, 大型 化优势 突出。 ( .  ̄- ,- r q 讯) 圆
硅磷铝酸盐及有关结晶氧化物[发明专利]
![硅磷铝酸盐及有关结晶氧化物[发明专利]](https://img.taocdn.com/s3/m/0570cc94f242336c1fb95e3a.png)
专利名称:硅磷铝酸盐及有关结晶氧化物
专利类型:发明专利
发明人:埃里克·杰勒德·德鲁安尼,罗兰德·冯·鲍尔木斯申请号:CN85104928
申请日:19850627
公开号:CN85104928A
公开日:
19861224
专利内容由知识产权出版社提供
摘要:硅磷铝酸盐是由包括氧化硅源、氧化铝源和氧化磷源以及一种合适的定向剂的二相反应混合物中结晶出来的。
这种硅磷铝酸盐的硅、磷和铝可以各自任意地由其他正四价态、正五价态和正三价态的元素所替代。
所生成的结晶氧化物具有离子交换特性,并易于转变成具有催化活性的物质。
申请人:美孚石油公司
地址:美国弗吉尼亚州22037-0001费尔法克斯·盖洛斯路3225号
国籍:US
代理机构:中国国际贸易促进委员会专利代理部
更多信息请下载全文后查看。
石油烃类污染物在地下水中自然衰减特性

石油烃类污染物在地下水中自然衰减特性
张渤;韩洁
【期刊名称】《重庆环境科学》
【年(卷),期】2002(024)005
【摘要】污染物在地下水中的自然衰减是目前解决地下水污染问题的一个重要的研究领域,是地下水污染生物修复的基础课题.本文以地下水中的常见污染物石油烃为对象,着重阐述该物质在地下水中的自然衰减特性.根据所获取的国内外资料,介绍了其自然衰减的三种动力学方程:Monod动力学方程,一级衰减动力学方程和瞬时反应动力学方程,并且对于石油烃的自然衰减速率进行了详细的描述,为以后的研究提供参考.
【总页数】4页(P35-37,54)
【作者】张渤;韩洁
【作者单位】清华大学核研院,北京,100084;清华大学核研院,北京,100084
【正文语种】中文
【中图分类】X132
【相关文献】
1.石油烃类污染物在地下水中自然衰减特性 [J], 张渤;韩洁
2.改性凹凸棒土对地下水中石油烃类吸附的影响 [J], 韩志勇;唐凤琳;鹿玲;杜旺兵;苏思;陆妍
3.地下水渗流中高铁酸钾氧化去除石油烃类污染物的试验研究 [J], 张兴;杨敏;柯佳闻;邵冰阳;王志科;林柯帆;官诗奇;李佩佩
4.缺氧地下水中石油烃类去除的原位生物修复 [J], 孙瑞;殷瑶
5.浅层地下水中石油类污染物监测自然衰减(MNA)预测与适宜性评价研究 [J], 王威;陈社明;马震;陈彭
因版权原因,仅展示原文概要,查看原文内容请购买。
Soil Microbial Community Dynamics
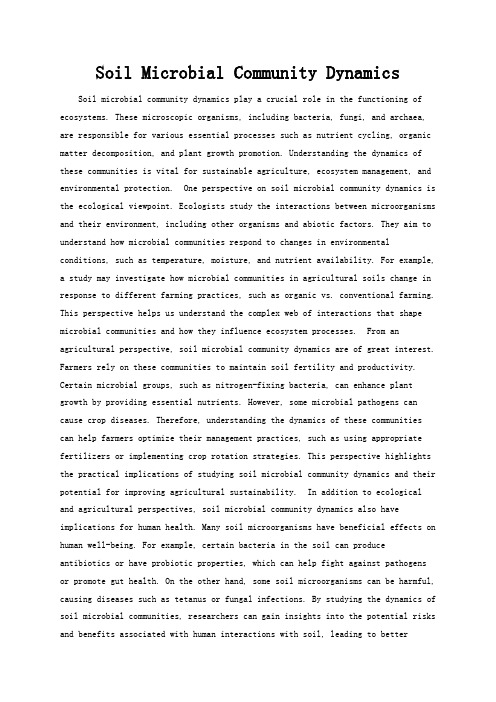
Soil Microbial Community Dynamics Soil microbial community dynamics play a crucial role in the functioning of ecosystems. These microscopic organisms, including bacteria, fungi, and archaea, are responsible for various essential processes such as nutrient cycling, organic matter decomposition, and plant growth promotion. Understanding the dynamics of these communities is vital for sustainable agriculture, ecosystem management, and environmental protection. One perspective on soil microbial community dynamics is the ecological viewpoint. Ecologists study the interactions between microorganisms and their environment, including other organisms and abiotic factors. They aim to understand how microbial communities respond to changes in environmental conditions, such as temperature, moisture, and nutrient availability. For example, a study may investigate how microbial communities in agricultural soils change in response to different farming practices, such as organic vs. conventional farming. This perspective helps us understand the complex web of interactions that shape microbial communities and how they influence ecosystem processes. From an agricultural perspective, soil microbial community dynamics are of great interest. Farmers rely on these communities to maintain soil fertility and productivity. Certain microbial groups, such as nitrogen-fixing bacteria, can enhance plant growth by providing essential nutrients. However, some microbial pathogens can cause crop diseases. Therefore, understanding the dynamics of these communities can help farmers optimize their management practices, such as using appropriate fertilizers or implementing crop rotation strategies. This perspective highlights the practical implications of studying soil microbial community dynamics and their potential for improving agricultural sustainability. In addition to ecological and agricultural perspectives, soil microbial community dynamics also have implications for human health. Many soil microorganisms have beneficial effects on human well-being. For example, certain bacteria in the soil can produceantibiotics or have probiotic properties, which can help fight against pathogens or promote gut health. On the other hand, some soil microorganisms can be harmful, causing diseases such as tetanus or fungal infections. By studying the dynamics of soil microbial communities, researchers can gain insights into the potential risks and benefits associated with human interactions with soil, leading to betterpublic health practices and policies. Socio-economic factors also come into play when considering soil microbial community dynamics. For instance, the degradation of soil microbial communities due to intensive farming practices or pollution can have significant economic consequences. Reduced soil fertility and productivitycan lead to decreased agricultural yields and increased reliance on synthetic fertilizers, which can be costly and environmentally damaging. Moreover, the loss of beneficial soil microorganisms may also impact industries that rely onmicrobial products, such as pharmaceuticals or bioremediation. Therefore, understanding and managing soil microbial community dynamics is crucial for sustainable development and economic stability. Finally, it is essential to acknowledge the emotional connection that humans have with soil microbial community dynamics. Soil is not just a medium for plant growth; it is a living ecosystem that supports life on Earth. The intricate relationships between microorganisms and the soil environment are awe-inspiring and remind us of the interconnectedness of all living things. Moreover, the potential for harnessingthe power of soil microorganisms to address environmental challenges, such as climate change or pollution, can evoke feelings of hope and excitement. Bystudying soil microbial community dynamics, we can deepen our connection with the natural world and appreciate the beauty and complexity of these hidden ecosystems. In conclusion, soil microbial community dynamics are a fascinating and essential field of study with multiple perspectives. From an ecological viewpoint, understanding the interactions between microorganisms and their environment helps us unravel the complexity of microbial communities and their role in ecosystem functioning. From an agricultural perspective, studying soil microbial communities can lead to improved farming practices and sustainable food production. The implications for human health highlight the potential risks and benefitsassociated with soil microorganisms. Socio-economic factors emphasize the economic consequences of soil degradation and the importance of managing microbial communities for sustainable development. Finally, the emotional connection that humans have with soil microbial community dynamics reminds us of the wonder and interconnectedness of the natural world. Overall, studying soil microbialcommunity dynamics is crucial for the well-being of ecosystems, agriculture, human health, and our own connection with the Earth.。
地下水风险评估计算方法
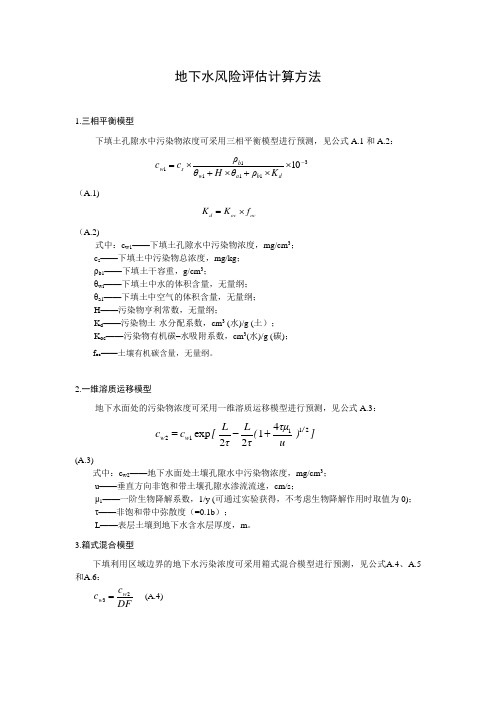
地下水风险评估计算方法1.三相平衡模型下填土孔隙水中污染物浓度可采用三相平衡模型进行预测,见公式A.1和A.2:31111110-⨯⨯+⨯+⨯=d b a w b s w K ρθH θρc c(A.1) oc oc d f K K ⨯=(A.2)式中:c w1——下填土孔隙水中污染物浓度,mg/cm 3;c s ——下填土中污染物总浓度,mg/kg ;ρb1——下填土干容重,g/cm 3;θwl ——下填土中水的体积含量,无量纲;θa1——下填土中空气的体积含量,无量纲;H ——污染物亨利常数,无量纲;K d ——污染物土-水分配系数,cm 3 (水)/g (土);K oc ——污染物有机碳–水吸附系数,cm 3(水)/g (碳);f oc ——土壤有机碳含量,无量纲。
2.一维溶质运移模型地下水面处的污染物浓度可采用一维溶质运移模型进行预测,见公式A.3: ])u τμ(τL τL [c c /w w 211124122exp +-=(A.3)式中:c w2——地下水面处土壤孔隙水中污染物浓度,mg/cm 3;u ——垂直方向非饱和带土壤孔隙水渗流流速,cm/s ;μ1——一阶生物降解系数,1/y (可通过实验获得,不考虑生物降解作用时取值为0); τ——非饱和带中弥散度(=0.1b );L ——表层土壤到地下水含水层厚度,m 。
3.箱式混合模型下填利用区域边界的地下水污染浓度可采用箱式混合模型进行预测,见公式A.4、A.5和A.6: DFc c w w 23= (A.4)gw gwgw W I δU DF ⨯⨯+=1 (A.5) )))B U IW ((B W .(B,δgw gw gw gw ⨯⨯-+⨯+⨯=exp 1105830min (A.6)式中:c w3——经混合稀释后地下水中污染物浓度,mg/cm 3; DF ——地下水混合稀释因子,无量纲; U gw ——地下水流速,cm/a ; δgw ——地下水混合层厚度,cm ; W gw ——平行于地下水流向的污染土壤长度,cm ; I ——土壤中水的入渗率,cm/a ; B ——含水层厚度,cm 。
中国科学院成都有机化学公司天然气转化催化剂项目通过验收

中国科学院成都有机化学公司天然气转化催化剂项目通过验收佚名
【期刊名称】《工业催化》
【年(卷),期】2006(14)7
【摘要】中国科学院成都有机化学公司与川化集团合作,在国内率先开展了Brown流程天然气低水碳比造气催化技术的开发,形成了预转化、一段转化、二段转化、高温变换和低温交换五大催化核心技术,各段低水碳比转化催化剂已实现工业应用。
2005年,中国科学院成都有机化学公司与中国石化大庆石化分公司合作,成功开发出新型低碳水比一段转化催化剂。
【总页数】1页(P22-22)
【关键词】一段转化催化剂;中国科学院;化学公司;天然气;通过验收;有机;成都;大庆石化分公司;项目;低水碳比
【正文语种】中文
【中图分类】TQ113.247
【相关文献】
1.耕耘于化学的沃野培育催化剂之花——记中国科学院成都有机化学研究所陶家林研究员 [J],
2.成都有机化学公司建成100t/a甲醇氧化羰化催化剂装置 [J], 孙可华
3.成都有机化学公司天然气转化催化剂项目通过验收 [J],
4.成都有机化学公司和川化集团公司两个项目通过鉴定 [J],
5.中国科学院广州化学公司有机锑热稳定剂通过验收 [J],
因版权原因,仅展示原文概要,查看原文内容请购买。
橡树岭国家实验室研发出适应寒冷气候的高效空气源热泵
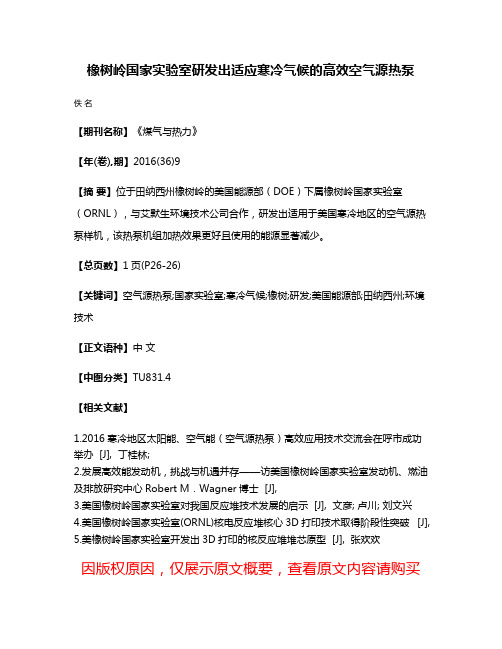
橡树岭国家实验室研发出适应寒冷气候的高效空气源热泵
佚名
【期刊名称】《煤气与热力》
【年(卷),期】2016(36)9
【摘要】位于田纳西州橡树岭的美国能源部(DOE)下属橡树岭国家实验室(ORNL),与艾默生环境技术公司合作,研发出适用于美国寒冷地区的空气源热泵样机,该热泵机组加热效果更好且使用的能源显著减少。
【总页数】1页(P26-26)
【关键词】空气源热泵;国家实验室;寒冷气候;橡树;研发;美国能源部;田纳西州;环境技术
【正文语种】中文
【中图分类】TU831.4
【相关文献】
1.2016寒冷地区太阳能、空气能(空气源热泵)高效应用技术交流会在呼市成功
举办 [J], 丁桂林;
2.发展高效能发动机,挑战与机遇并存——访美国橡树岭国家实验室发动机、燃油及排放研究中心Robert M.Wagner博士 [J],
3.美国橡树岭国家实验室对我国反应堆技术发展的启示 [J], 文彦; 卢川; 刘文兴
4.美国橡树岭国家实验室(ORNL)核电反应堆核心3D打印技术取得阶段性突破 [J],
5.美橡树岭国家实验室开发出3D打印的核反应堆堆芯原型 [J], 张欢欢
因版权原因,仅展示原文概要,查看原文内容请购买。
- 1、下载文档前请自行甄别文档内容的完整性,平台不提供额外的编辑、内容补充、找答案等附加服务。
- 2、"仅部分预览"的文档,不可在线预览部分如存在完整性等问题,可反馈申请退款(可完整预览的文档不适用该条件!)。
- 3、如文档侵犯您的权益,请联系客服反馈,我们会尽快为您处理(人工客服工作时间:9:00-18:30)。
Soil Carbon and Nitrogen Dynamics Following Application of Pig Slurry for the 19thConsecutive Year:I.Carbon Dioxide Fluxes and Microbial Biomass CarbonPhilippe Rochette,*Denis A.Angers,and Denis Coˆte ´ABSTRACTfrom storage and handling conditions (Kirchmann and Lunvall,1993).It is not clear whether information ob-Agricultural soils often receive annual applications of manure fortained from laboratory incubations can be directly ap-long periods.The objective of this study was to quantify the effects of 19consecutive years of pig (Sus scrofa )slurry (PS)application plied to field conditions where many factors simultane-on CO 2emissions and soil microbial biomass.Soil temperature,soil ously affect decomposition.We are not aware of moisture,and extractable soil C were also determined to explain the experimental data describing decomposition rates of pig variations in CO 2emissions and soil microbial biomass.Long-term slurry under field conditions.(19yr)treatments were 60(PS60)and 120Mg ha Ϫ1yr Ϫ1(PS120)of On many hog farms,the land area required to dispose pig slurry and a control receiving mineral fertilizers at a dose of 150of the volume of slurry exceeds the land available.It is kg ha Ϫ1yr Ϫ1each of N,P 2O 5,and K 2O.Very high CO 2emissions (up to therefore common that soils receive slurry every year 1.5mg CO 2m Ϫ2s Ϫ1)occurred during the first 2d after PS application.for long periods of time.The objective of this study was Following that peak,decomposition of PS was rapid,with one-half to quantify the effects of 19consecutive years of pig the total emissions occurring during the first week after slurry applica-tion.The rapid initial decomposition was exponential and was attrib-slurry application on CO 2emissions and soil microbial uted to the decomposition of the labile fraction of the slurry C.The biomass.Soil temperature,soil moisture,and extract-second phase was linear and much slower and probably involved able soil C were also determined to explain the varia-more recalcitrant C material.Cumulative annual decomposition was tions in CO 2emissions and soil microbial biomass.proportional to the application rate,with 769and 1658kg C ha Ϫ1lost from the 60and 120Mg ha Ϫ1doses,respectively.Pig slurry application MATERIALS AND METHODScaused a rapid increase in soil microbial biomass (from ≈100to up to 370mg C kg Ϫ1soil),which coincided with a peak in the concentra-The experiment was conducted from 30May 1997to 27tion of extractable C and in CO 2emissions.Field estimates of the May 1998at the St-Lambert Research Farm of the Que ´bec microbial specific respiratory activity suggested that the difference in Ministry of Fisheries,Agriculture,and Food near Que´bec soil respiration between the two slurry treatments was due to differ-City,Canada (46Њ05ЈN,71Њ02ЈW,altitude 110m).The soil ences in the size of the induced microbial biomass rather than to was a Le Bras loam (frigid Aeric Haplaquept)with 0.31g differences in specific activity.sand,0.42g silt,and 0.27g clay g Ϫ1soil.Soil C and N contents averaged across treatments were 19.9and 1.2g kg Ϫ1,respec-tively,prior to the 1997slurry application.Measurements were made on plots that were initiated in 1979to assess the impact Decomposition of soil organic matter (SOM)af-of long-term application of PS on silage maize (Zea mays L.)fects the dynamics of various nutrients such as N yields and soil properties (N’dayegamiye and Coˆte ´,1989).and P,influences soil physical and chemical properties,Tillage operations were one pass of chisel plow in the fall and and directly impacts the atmospheric concentration of two passes of field cultivator in the spring prior to planting.The CO 2,a potent greenhouse gas (Intergovernmental Panel treatments consisted of annual applications of either mineral on Climate Change,1996;Baldock and Nelson,2000).fertilizer (150kg ha Ϫ1NH 4NO 3–N,150kg ha Ϫ1P 2O 5,150kg Animal wastes are a significant source of organic C for ha Ϫ1K 2O)(control)broadcasted prior to planting (27May agricultural soils.Hog industry produces ≈8.8million1997),or pig slurry at rates of 60(PS60)or 120Mg ha Ϫ1(PS120)banded at the six-to eight-leaf stage (30June 1997)m 3of slurry annually in the province of Que´bec,Canada repeated three times in randomized blocks.In 1997,the slurry (Trudelle,1996).Most of this slurry is applied to agricul-was from a commercial hog and sow operation and containedtural soils either in spring or fall,where it is subjected to 2.1kg m Ϫ3total N,0.99kg m Ϫ3NH ϩ4–N,1.15kg m Ϫ3P,and decomposition by the soil biota.Application of animal 1.29kg m Ϫ3Ca.Maize was planted on 28May 1997at 76000slurry usually increases the size of the soil microflora seeds ha Ϫ1and 0.75-m interrows.Slurry was applied in 0.6-both in the short (Opperman et al.,1989)and long termm-wide bands and shallow-incorporated (0.075m)in each (N’dayegamiye and Coˆte ´,1989;Rochette and Gre-interrow using a liquid manure spreader equipped with drop gorich,1998).tubes and tines mounted on a boom at the rear of the spreaderDecomposition of pig slurry in soils has been studied (Jokela and Coˆte ´,1994).Average maize aboveground dry in laboratory rge variations in decompo-matter yields between 1990and 1995were 7.1Mg ha Ϫ1for sition rates have been observed between slurries (Den-the control,7.9Mg ha Ϫ1for PS60,and 9.3Mg ha Ϫ1for PS120.dooven et al.,1998a,1998b;Morvan and Leterme,1999)(Table 1).These differences have been related to varia-Soil Surface Carbon Dioxide Fluxestions in slurry composition resulting,among other fac-In situ CO 2fluxes were measured by the dynamic closed tors,from animal type (Morvan and Leterme,1999)andchamber method detailed by Rochette et al.(1997)and briefly described as follows.Three acrylic frames (0.60by 0.60m;Philippe Rochette and Denis A.Angers,Soils and Crops Research 0.14-m height;6.35-mm wall thickness)were inserted to a and Development Centre,Agriculture and Agri-Food Canada,2560depth of 0.10m in each plot on 29May 1997.The framesHochelaga Blvd.,Sainte-Foy,QC,Canada,G1V 2J3;Denis Coˆte ´,Institut de recherche et de de ´veloppement en agroenvironnement,Complexe Scientifique,2700Einstein St.,Sainte-Foy,QC,Canada,Abbreviations:MBC,microbial biomass C;PS,pig slurry;PS60,appli-G1P 3W8.Received 1July 1999.*Corresponding author (rochettep@cation of pig slurry at 60Mg ha Ϫ1yr Ϫ1;PS120,application of pig em.agr.ca).slurry at 120Mg ha Ϫ1yr Ϫ1;SOM,soil organic matter;SRA,specific respiratory activity.Published in Soil Sci.Soc.Am.J.64:1389–1395(2000).13891390SOIL SCI.SOC.AM.J.,VOL.64,JULY–AUGUST 2000Table 1.Summary of the dynamics of liquid hog manure decomposition in laboratory incubations.Temperature Duration C loss Remarks Reference؇C d %of added C162417–4313pig slurries Morvan and Leterme (1999)252860Dendooven et al.(1998a)257075Fresh Bernal and Kirchmann (1992)257023Aerobic Bernal and Kirchmann (1992)2570105Anaerobic Bernal and Kirchmann (1992)257065FreshKirchmann and Lundvall (1993)257048AnaerobicKirchmann and Lundvall (1993)25155Dendooven et al.(1998b)2223044Saviozzi et al.(1997)232847Castellanos and Pratt (1981)completely covered the width of the slurry application band late the MBC (Wu et al.,1990).Specific respiratory activity of soil microbial biomass was calculated as the quotient of between maize rows and were left at the same locations for the duration of the experiment.When slurry was applied,the respired CO 2to MBC.For that purpose,MBC was expressed in grams of C per square meter using bulk densities measured tines were lifted to clear the frame and the slurry was deposited at the surface and immediately mixed into the top 7.5cm of soil in 1994(1.24g cm Ϫ3in the control,1.24g cm Ϫ3in the PS60,and 1.30g cm Ϫ3in the PS120plots [M.A.Bolinder,1995,with hand tools.The frames height was measured at regular intervals during the experiment (48measuring points per personal communication]).A fraction of the soil samples was air dried and sieved at frame)to account for variations in headspace due to soil settling.2mm for the determination of soil pH (CaCl 2,0.01M ).Total C and N contents were determined on a Leco CNS 1000The CO 2flux measuring system was equipped with an LI-6200CO 2analyzer (LI-COR,Lincoln,NE)and a 0.15-m-(LECO,St.Joseph,MI)on finely ground (0.05mm)soil samples.high square plexiglass chamber covering the same area as the frames.During each CO 2flux measurement,the chamber was fixed to a frame and the CO 2concentration inside the chamber RESULTS AND DISCUSSIONwas measured once every second during four 20-s successive periods.The mean CO 2flux (F CO2)on each frame was calcu-Soil temperature at 10cm was not affected by the lated as follows:treatments (Fig.1a).In 1997,temperature increased in June,peaked in July,was slightly lower in August (probably as a result of the shading by the maize can-F CO2ϭ͚31΄C i ϩ1ϪC i t i ϩ1Ϫt i m m m v VA΅3[1]opy),and gradually decreased in September and Octo-ber.In 1998,soil temperature increased rapidly in May where C i is the mean CO 2concentration during period i (L due to a warm and dry spring.Soil moisture was similar L Ϫ1);t i is the mean time of period i (s);m m and m v are the between treatments and was averaged across treatments molecular mass and volume of CO 2,respectively;V is the total for each depth (Fig.1b).Soil moisture below 5cm re-system volume including chamber,frame,and gas analyzer mained between 0.20and 0.35m 3m Ϫ3during most of tubings (≈68L depending on frame height);and A is the area the study.Water contents above 0.35m 3m Ϫ3were mea-covered by the chamber (0.36m 2).sured in early July 1997following slurry application and Soil temperature at a depth of 10cm inside each frame and abundant rainfalls in the fall of 1997and in the spring volumetric water content at depths of 5,10,20,and 40cm of 1998(Fig.1b).(one profile per plot)were measured at the time of the CO 2flux measurements.Soil temperature and soil moisture were measured using copper-constantan thermocouples and time Carbon Dioxide Emissions in the Control Plotsdomain reflectometry probes,respectively.The effects of Soil surface CO 2fluxes in the control plots receiving treatments were analyzed at each date using a two-way analysis of variance (SAS Institute,1989).mineral fertilizer were exceptionally low throughout the experiment (Fig.2).Values above 0.05mg m Ϫ2s Ϫ1were measured only between 15July and 15September when Soil Analysesthey reached a maximum of 0.09mg m Ϫ2s Ϫ1.In compari-Soil samples were collected from the 15-cm surface soil son,Gregorich et al.(1998)and Rochette and Gregorich layer 19times during the experiment.Samples were sieved in (1998)reported values up to 0.21mg m Ϫ2s Ϫ1for miner-the field at 6mm and stored immediately at 3ЊC.Soil microbial ally fertilized grain maize fields in Ottawa,Canada.Low biomass C (MBC)measurements were carried out within 24h F CO2in the control plots has to result from the low of sampling using the chloroform fumigation–extraction tech-nique (Wu et al.,1990).Two 50-g subsamples of field-moist activity of one or both main CO 2sources:the oxidation soils were placed in 100-mL beakers.One subsample was of soil C by heterotrophs and the root–rhizosphere res-fumigated for 24h at room temperature in a vacuum desiccator piration.The activity of heterotrophs is driven by the containing 25mL of CHCl 3.The other subsample was kept availability of substrates and is modulated by the envi-in the dark at 3ЊC for 24h.Both fumigated and nonfumigated ronmental conditions.Under conditions of abundant soils were extracted with 100mL of 0.25M K 2SO 4.After substrates and sufficient moisture,respiration is strongly shaking for 1h on an oscillating shaker,the suspensions were correlated with soil temperature (Rochette and Gre-centrifuged at 1000g and filtered.The organic C content of gorich,1998).In the control plots,respiration values the extracts was determined by UV-persulfate oxidation on were weakly correlated with soil temperature (r ϭ0.35,a DC-180Carbon Analyzer (Dorhman Co.,Santa Clara,CA).An extraction efficiency (K ec factor)of 0.45was used to calcu-P Ͼ0.1),thereby suggesting that respiration was limitedROCHETTE ET AL:CARBON DYNAMICS AFTER19YEARS OF PIG SLURRY APPLICATION1391Fig.1.Temporal variations of(a)soil temperature at a depth of10cm for maize soils amended with mineral fertilizer(control)and pig slurry at60(PS60)and120Mg haϪ1(PS120)and(b)soil moisture at depths of5,10,20,and40cm averaged across treatments.by the availability of organic substrates and was domi-tend to converge,so that the amount of stored C is nated by root–rhizosphere respiration.relatively static(Janzen et al.,1997).After19yr of The amount of organic C that is oxidized in the soil uniform tillage and cropping practices,one can assume annually by heterotrophic respiration can be estimated.that the soil C is near equilibrium and that the amount With time,the rates of input and loss of C from soil of C that is oxidized annually is approximately equal toFig.2.Temporal variations of CO2flux from maize soils amended with mineral fertilizer(control)and pig slurry at60(PS60)and120Mg haϪ1 (PS120).Mineral fertilizer was applied on29May and pig slurry on30June1997.1392SOIL SCI.SOC.AM.J.,VOL.64,JULY–AUGUST2000Fig.3.Slurry-induced CO2–C losses from maize soils amended with pig slurry at60(PS60)and120Mg haϪ1(PS120).Pig slurry was applied on30June1997.the amount of C that is returned to the soil.The average during these first2d.Carbon dioxide is produced in aboveground yield of the fertilized silage maize(con-slurry during storage by the hydrolysis of urea and the trol)between1990and1995was7.1Mg dry matter anaerobic decomposition of volatile fatty acids(Som-mer and Husted,1995).The CO2in solution forms pH-haϪ1(Coˆte´et al.,1996).Neglecting the contribution ofstubble and assuming a root/shoot ratio of0.2and a dependent chemical equilibriums(carbonates)with plant residue C content of40%,570kg C haϪ1wereother species such as NH3(Sommer and Husted,1995). returned as crop residues annually.The oxidation of The pH of the slurry was≈8,while that of the surface this amount of C in the growing season(150d)corre-soil was5.69Ϯ0.13in PS60and5.79Ϯ0.15in the sponds with an average flux of0.016mg CO2mϪ2sϪ1.PS120plots following PS application(1July).These Therefore,the low return of residue C to soil in thisvalues are lower than the threshold(7.2)below which continuous silage maize system can alone explain the ammonium carbonates are dissociated and CO2is re-leased.Ge´nermont(1996)also reported very large car-low F CO2measured in the control plots.Also,consideringthat root–rhizosphere respiration of a maize crop in bonate-induced CO2emissions(1.4mg CO2mϪ2sϪ1) eastern Canada is insignificant prior to1July(Rochettefollowing application of132m3haϪ1of cattle(Bos tau-and Flanagan,1997;Rochette et al.,1999b),most of the rus)slurry under field conditions.respired CO2–C that occurred in June arose from soilFollowing this initial burst,an adjustment phase of C oxidation.Cumulative CO2–C emitted during that≈20d was observed during which the differences be-tween manured and control plots decreased gradually. period corresponded with234kg C haϪ1or41%of theannual return of crop residue C.This indicates that the We postulate that this period reflected the time during contribution of soil C oxidation during the remainingwhich the soil heterotrophs were using up the amount part of the growing season was even less than0.016mg of readily decomposable organic substrates of the PS. CO2mϪ2sϪ1and that F CO2measured from July to the endFinally,the adjustment phase was followed by a period of the season probably reflected the root–rhizosphere of small but fairly constant differences between the ma-nured plots and the control plots.During this period, respiration of the maize crop.This is supported by theobservation that the temporal pattern of F CO2was similar more recalcitrant organic substrates were probably de-composed.The temporal pattern of F CO2from late July to that of the maize crop growth after1July.to the end of1997was the same on manured and control Carbon Dioxide Emissions in the Manured Plots plots,indicating that the temporal variations of the fluxwere governed by factors other than the addition of Values of F CO2on the manured and control plots wereslurry,probably the root–rhizosphere respiration of the similar early in the season when significant differencesmaize crop.(PϽ0.05)were measured only on the last day prior toapplication of PS(Fig.2).Thus,addition of PS in the Slurry-Induced Carbon Dioxide-Carbon Losses 18previous years had little impact on the oxidation ofsoil C early in1997.In contrast,significant differences Slurry-induced CO2–C emissions were estimated bysubtracting F CO2on the control plots from F CO2on the (PϽ0.05)were observed on14of the18sampling datesfollowing the application of PS in1997.The increase in manured plots.For this purpose,only the CO2emissions F CO2resulting from the addition of PS could be split intofrom within the slurry application band were considered. three periods.First,the fluxes were increased by a factor Cumulative slurry-induced CO2–C losses were then cal-culated by linearly interpolating CO2emissions between of10to30compared with background values duringthe first2d following application.These values are very sampling dates,assuming that measurements made be-tween0900and1200h were a good estimator of average high,and CO2release from displacement of physico-chemical equilibrium probably dominated the emissions daily F CO2.The early PS-induced CO2kinetics were simi-ROCHETTE ET AL:CARBON DYNAMICS AFTER19YEARS OF PIG SLURRY APPLICATION1393Fig.4.Temporal variations of soil microbial biomass C for maize soils amended with mineral fertilizer(control)and pig slurry at60(PS60)and 120Mg haϪ1(PS120).Mineral fertilizer was applied on29May and pig slurry on30June1997.lar for both slurry doses,with50,60,and70%of the Microbial Biomass and Activitytotal emissions lost after11,21,and40d,respectively.Despite an apparently higher(50%)MBC content After70d,87%of the total PS-induced CO2was lost in the two PS-amended soils than in the control,the in the PS60plots and82%in the PS120plots.Approxi-differences in MBC prior to the annual PS application mately one-half of the losses occurred during the first(25June)were not statistically significant at PϽ0.05. week after slurry addition(Fig.3).Total losses in1997This indicates that there were no significant long-term from the PS60plots(769kg C haϪ1)were46%of those(18yr)residual effects of PS on MBC.The long-term measured from the PS120plots(1658kg C haϪ1),indi-effect of PS on total C content was not significant either cating a linear response of C oxidation to the amount(17.9,20.6,and20.8g C kgϪ1soil for the control,PS60, of substrate added and suggesting that there were no and PS120,respectively).The field variation in total C physical or chemical limitations to increased microbial content(coefficient of variation of24%,nϭ9on25 activity with increased amount of PS added.Gregorich June)is often high to the point that management-in-et al.(1998)reported a decreasing response of CO2duced differences in soil C content may be difficult to emissions with increasing dose of solid cattle manure detect(Paustian et al.,1997).under field conditions.Differences between the CO2–C The1997annual PS application caused a rapid in-emissions induced by solid and liquid manures can be crease in MBC in early July,which was linear with the explained at least partly by the easier access to the highly rate of application(Fig.4).Laboratory studies have diluted substrates in PS than in solid manure,which shown this rapid increase in MBC immediately follow-usually forms clods in which the inner portions are physi-ing PS application(Saviozzi et al.,1997).The peak in cally protected from the attack by decomposers.MBC lasted only for a few days but the difference be-Fig.5.Temporal variations of extractable C for maize soils amended with mineral fertilizer(control),and pig slurry at60(PS60)and120Mg haϪ1(PS120).Mineral fertilizer was applied on29May and pig slurry on30June1997.1394SOIL SCI.SOC.AM.J.,VOL.64,JULY–AUGUST 2000Fig.6.Temporal variations of specific activity of soil microbial biomass for maize soils amended with mineral fertilizer (control)and pig slurry at 60(PS60)and 120Mg ha Ϫ1(PS120).Mineral fertilizer was applied on 29May and pig slurry on 30June 1997.MBC is microbial biomass C.(Rochette et al.,1999a).In our study,CO 2fluxes and tween the two amended treatments lasted for ≈2mo.MBC were influenced by three C sources:native soil The peak in early July,right after PS application,coin-C,plant activity,and PS.In order to isolate the effect cided with the peak of respiration activity (Fig.2).Jen-of PS from the contribution of plant roots and native sen et al.(1997)studied the dynamic response of MBC soil C to respiration and microbial biomass,we sub-and field respiration to rape (Brassica napus L.var.tracted the values of the control from the slurry treat-napus )straw application and found that MBC did not ments.The values of SRA induced by PS ranged from contribute significantly in explaining the variability of zero or slightly negative to 0.37g CO 2–C g MBC d Ϫ1soil surface CO 2flux.A similar conclusion was reached (Fig.6),which is in the range of values found under by Rochette and Gregorich (1998)using solid cattle laboratory conditions (Santruckova and Straskraba,manure.Our results are in agreement with those obser-1991).vations,except for the period immediately following PS Specific respiratory activity has been used to charac-application when both CO 2flux and MBC increased for terize the effect of disturbance on microbial biomass a short time.The difference between our study and the and its activity.In general,SRA increases following two others may be that PS contains high levels of soluble disturbances such as rewetting of dry soil,herbicide C and this was reflected in an increase in extractable C application,acidification or substrate addition,and de-in the soil following PS application as well (Fig.5).clines following recovery from disturbance (Wardle and Differences in extractable C between treatments were Ghani,1995).The decline would be due to approaching only significant for a few days after PS application (P equilibrium conditions,which would cause the mi-Ͻ0.01on 2and 8July).It is also possible that part of croflora to become more efficient at conserving C.The the increase in MBC measured a few days following PS ratio rapidly increased and declined after PS applica-application be attributable to the presence of microbes tions (Fig.6),which is characteristic of an easily decom-in the applied manure as proposed for compost by Per-posable substrate (Wardle and Ghani,1995).As men-ucci (1990).tioned above,the increase in CO 2flux in the first 2d After a low in mid July,MBC as well as extractable after PS application was probably not of biological ori-C started to increase until early September in all treat-gin and therefore should not be considered in the discus-ments.The high value at that date also corresponded sion on specific activity.However,the high values on with the highest gravimetric soil water content (data not 8July suggest that the higher soil respiration in both shown).Overall,a weak but significant correlation was manured plots (Fig.2)was not only due to the increased found between MBC and gravimetric soil water content size of the microflora (Fig.4)but also its activity.It is (r ϭ0.45;P Ͻ0.01).In October 1997and in the spring noteworthy that both slurry treatments showed the same of 1998,MBC was similar in both slurry treatments as SRA,which indicates a similar efficiency at decompos-it had been similar in the spring of 1997before slurry ap-ing PS and an adaptation of the microflora to the amount plication.of substrate.This observation also suggests that the dif-Higher respiration following addition of PS can be ference in total respiration between the two PS treat-the result of an increase in microbial biomass or its ments (Fig.2)was due to a difference in the size of the activity.Specific respiratory activity (SRA)of the soil microbial biomass and not to a difference in its specific microbial biomass,which is the ratio of the respiratory activity.The SRA quickly decreased after the peak to reach a temporary equilibrium level at 0.02to 0.04g activity to microbial biomass,was calculated to de-termine the relative contribution of these two factors.CO 2–C g MBC d Ϫ1.After 50to 60d following PS applica-tion,the quotient was close to zero,which suggests that Specific respiratory activity has been determined under laboratory incubation conditions (Santruckova and the microflora induced by the addition of PS was not active anymore.Straskraba,1991)but rarely under field conditionsROCHETTE ET AL:CARBON DYNAMICS AFTER 19YEARS OF PIG SLURRY APPLICATION1395Soil carbon dynamics in Canadian agroecosystems.p.57–80.In R.CONCLUSIONSLal et al.(ed.)Soil processes and the carbon cycle.CRC Press,Boca Raton,FL.Our approach allowed the characterization of the Jensen,L.S.,T.Mueller,J.Magid,and N.E.Nielsen.1997.Temporal mineralization of PS under field conditions.The decom-variation of C and N mineralization,microbial biomass and extract-position of PS was rapid,with one-half the total emis-able organic pools in soil after oilseed rape straw incorporation in sions occurring during the first week after slurry applica-the field.Soil Biol.Biochem.29:1043–1055.Jokela,W.,and D.Coˆte ´.1994.Options for direct incorporation of tion.The early decomposition was proportional to the liquid manure.p.201–215.In Proceedings of the liquid manure application rate.The rapid initial decomposition was application systems:Design,management and environmental as-exponential and was attributed to the decomposition of sessment.Rochester,NY.1–2Dec.1994.Northeast Regional Agric.the labile fraction of the slurry C.The second phase was Eng.Serv.,Ithaca,NY.linear and much slower,and probably involved more Kirchmann,H.,and A.Lunvall.1993.Relationship between N immo-bilization and volatile fatty acids in soil after application of pig recalcitrant C material.Pig slurry application caused a and cattle slurry.Biol.Fertil.Soils 15:161–164.rapid increase in soil microbial biomass that coincided Morvan,T.,and P.Leterme.1999.Short-term C and N transformations with an increase in the concentration of extractable C following pig and cattle slurry incorporation in soils.p.113–127In and in CO 2emissions.Field estimates of the microbial J.Martinez and M.N.Maudet (ed.)Ramiran 988th Int.Conf.on Management Strategies for Organic Waste Use in Agriculture.specific respiratory activity suggested that the difference Rennes,France.26–29May 1998.Cemagref Editions,Antony,in soil respiration between the two slurry treatments was France.due to differences in the size of the induced microbial N’dayegamiye,A.,and D.Coˆte ´.1989.Effect of long-term pig slurry biomass and not to its specific activity.and solid cattle manure application on soil chemical and biological properties.Can.J.Soil Sci.69:39–47.Opperman,M.H.,M.Wood,and P.J.Harris.1989.Changes in micro-ACKNOWLEDGMENTSbial populations following the application of cattle slurry to soil at two temperatures.Soil Biol.Biochem.21:263–268.This work was supported by the PERD-Climate Change Paustian,K.,H.P.Collins,and E.A.Paul.1997.Management controls program of Agriculture and Agri-Food Canada.We thank F.on soil carbon.p.15–49.In E.A.Paul et al.(ed.)Soil organic matter Gagne´,P.Jolicoeur,R.Baillargeon,N.Bissonnette,J.Lizotte,in temperate agroecosystems.CRC Press,Boca Raton,FL.and P.Drouin for assistance in soil respiration and microbial Perucci,P.1990.Effect of the addition of municipal solid-waste com-post on microbial biomass and enzyme activities in soil.Biol.Fertil.biomass measurements and plot maintenance.The numerous Soils.10:221–226.discussions and the review provided by Dr.M.H.Chantigny Rochette,P.,D.A.Angers,and L.B.Flanagan.1999a.Maize residue are also gratefully acknowledged.decomposition measurement using soil surface carbon dioxide fluxes and natural abundance of carbon 13.Soil Sci.Soc.Am.J.63:1385–1396.REFERENCESRochette,P.,B.Ellert,E.G.Gregorich,R.L.Desjardins,E.Pattey,Baldock,J.A.,and P.N.Nelson.2000.Soil organic matter.p.B-25–B-R.Lessard,and B.G.Johnson.1997.Description of a dynamic 84.In M.E.Sumner (ed.)Handbook of soil science.CRC Press,closed chamber for measuring soil respiration and its comparison Boca Raton,FL.with other techniques.Can.J.Soil Sci.77:195–203.Bernal,M.P.,and H.Kirchmann.1992.Carbon and nitrogen mineral-Rochette,P.,and L.B.Flanagan.1997.Quantifying rhizosphere respi-ization and ammonia volatilization from fresh,aerobically and an-ration in a corn crop under field conditions.Soil Sci.Soc.Am.aerobically treated pig manure during incubation with soil.Biol.J.61:466–474.Fertil.Soils 13:135–141.Rochette,P.,L.B.Flanagan,and E.G.Gregorich.1999b.Separating Castellanos,J.Z.,and P.F.Pratt.1981.Mineralization of manure nitro-soil respiration into plant and soil components using analyses of gen—Correlation with laboratory indexes.Soil Sci.Soc.Am.J.natural abundance of carbon 13.Soil Sci.Soc.Am.J.63:1207–1213.45:354–357.Rochette,P.,and E.G.Gregorich.1998.Dynamics of soil microbial Coˆte ´,D.,T.Sen Tran,and A.N’Dayegamiye.1996.Efficacite ´fertili-biomass C,soluble organic C and CO 2evolution after three years sante du lisier de porc e ´pandu en post-leve ´e du maı¨s.Agrosolof manure application.Can.J.Soil Sci.78:283–290.9:14–20.Santruckova,H.,and M.Straskraba.1991.On the relationship be-Dendooven,L.,E.Bonhomme,R.Merckx,and K.Vlassak.1998a.tween specific respiration activity and microbial biomass in soils.N dynamics and sources of N 2O production following pig slurry Soil Biol.Biochem.23:525–532.application to a loamy soil.Biol.Fertil.Soils 26:224–228.SAS Institute.1989.SAS/STAT user’s guide.Version 6.4th ed.Vol.Dendooven,L.,E.Bonhomme,R.Merckx,and K.Vlassak.1998b.1&2.SAS Inst.,Cary,NC.Injection of pig slurry and its effects on dynamics of nitrogen and Saviozzi,A.,R.Levi-Minzi,R.Riffaldi,and G.Vanni.1997.Role carbon in a loamy soil under laboratory conditions.Biol.Fertil.of chemical constituents of wheat straw and pig slurry on their Soils 27:5–8.decomposition in soil.Biol.Fertil.Soils 25:401–406.Ge´nermont,S.1996.Mode ´lisation de la volatilisation d’ammoniac Sommer,S.G.,and S.Husted.1995.The chemical buffer system in apre `s e ´pandage de lisier sur parcelle agricole.Ph.D.thesis.Univ.raw and digested animal slurry.J.Agric.Sci.124:45–53.Paul Sabatier,Toulouse,France.Trudelle,M.1996.Nature et composition des engrais de ferme et desGregorich,E.G.,P.Rochette,S.McGuire,B.C.Liang,and posts.p.136–138.In Colloque sur la fertilisation inte´gre ´e des 1998.Soluble organic carbon and carbon dioxide fluxes in maize sols—Compte-rendu des confe´rences.Conseil des Productions fields receiving spring-applied manure.J.Environ.Qual.27:Ve´ge ´tales du Que ´bec,Que ´bec,Canada.209–214.Wardle,D.A.,and A.Ghani.1995.A critique of the microbial meta-Intergovernmental Panel on Climate Change.1996.Agricultural op-bolic quotient (qCO 2)as a bioindicator of disturbance and ecosys-tions for mitigation of greenhouses gas emissions.p.744–771.In tem development.Soil Biol.Biochem.27:1601–1610.Climate change 1995—Impacts,adaptations and mitigation of Wu,J.,R.G.Joergensen,B.Pommerening,R.Chaussod,and P.M.change:Scientific-technical analyses.Camb.Univ.Press,Cam-Brookes.1990.Measurement of soil microbial biomass C by fumiga-bridge,UK.tion–extraction—An automated procedure.Soil Biol.Biochem.22:1167–1169.Janzen,H.H.,C.A.Campbell.E.G.Gregorich,and B.H.Ellert.1997.。