Gene duplication and hierarchical modularity in intracellular interaction networks
dna基因克隆及序列分析英语翻译
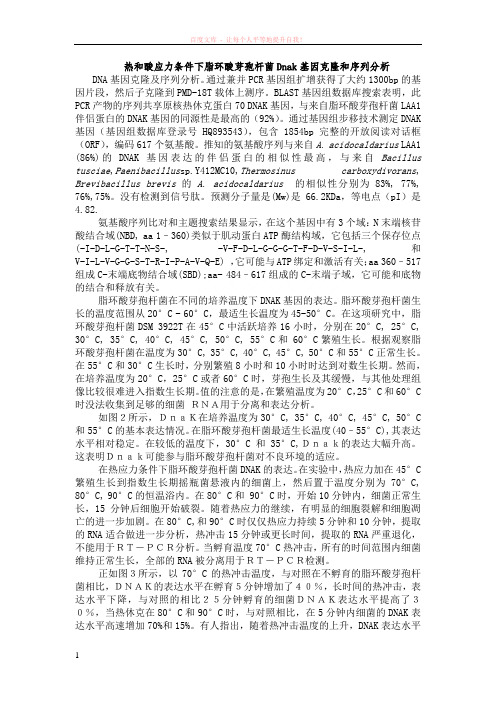
热和酸应力条件下脂环酸芽孢杆菌Dnak基因克隆和序列分析DNA基因克隆及序列分析。
通过兼并PCR基因组扩增获得了大约1300bp的基因片段,然后子克隆到PMD-18T载体上测序。
BLAST基因组数据库搜索表明,此PCR产物的序列共享原核热休克蛋白70 DNAK基因,与来自脂环酸芽孢杆菌LAA1伴侣蛋白的DNAK基因的同源性是最高的(92%)。
通过基因组步移技术测定DNAK 基因(基因组数据库登录号HQ893543),包含1854bp完整的开放阅读对话框(ORF),编码617个氨基酸。
推知的氨基酸序列与来自A. acidocaldarius LAA1 (86%)的DNAK基因表达的伴侣蛋白的相似性最高,与来自Bacillus tusciae,Paenibacillus sp.Y412MC10,Thermosinus carboxydivorans, Brevibacillus brevis 的 A. acidocaldarius 的相似性分别为83%, 77%, 76%,75%。
没有检测到信号肽。
预测分子量是(Mw)是66.2KDa,等电点(pI)是4.82.氨基酸序列比对和主题搜索结果显示,在这个基因中有3个域:N末端核苷酸结合域(NBD, aa 1–360)类似于肌动蛋白ATP酶结构域,它包括三个保存位点(-I-D-L-G-T-T-N-S-, -V-F-D-L-G-G-G-T-F-D-V-S-I-L-,和V-I-L-V-G-G-S-T-R-I-P-A-V-Q-E) ,它可能与ATP绑定和激活有关;aa 360–517组成C-末端底物结合域(SBD);aa- 484–617组成的C-末端子域,它可能和底物的结合和释放有关。
脂环酸芽孢杆菌在不同的培养温度下DNAK基因的表达。
脂环酸芽孢杆菌生长的温度范围从20°C - 60°C,最适生长温度为45-50°C。
在这项研究中,脂环酸芽孢杆菌DSM 3922T在45°C中活跃培养16小时,分别在20°C, 25°C, 30°C, 35°C, 40°C, 45°C, 50°C, 55°C和60°C繁殖生长。
(武汉大学)分子生物学考研名词汇总

(武汉大学)分子生物学考研名词汇总●base flipping 碱基翻出●denaturation 变性DNA双链的氢键断裂,最后完全变成单链的过程●renaturation 复性热变性的DNA经缓慢冷却,从单链恢复成双链的过程●hybridization 杂交●hyperchromicity 增色效应●ribozyme 核酶一类具有催化活性的RNA分子,通过催化靶位点RNA链中磷酸二酯键的断裂,特异性地剪切底物RNA分子,从而阻断基因的表达●homolog 同源染色体●transposable element 转座因子●transposition 转座遗传信息从一个基因座转移至另一个基因座的现象成为基因转座,是由转座因子介导的遗传物质重排●kinetochore 动粒●telomerase 端粒酶●histone chaperone 组蛋白伴侣●proofreading 校正阅读●polymerase switching 聚合酶转换●replication folk 复制叉刚分开的模板链与双链DNA的连接区●leading strand 前导链在DNA复制过程中,与复制叉运动方向相同,以5’-3’方向连续合成的链被称为前导链●lagging strand 后随链在DNA复制过程中,与复制叉运动方向相反的,不连续延伸的DNA链被称为后随链●Okazaki fragment 冈崎片段●primase 引物酶依赖于DNA的RNA聚合酶,其功能是在DNA复制过程中合成RNA引物●primer 引物是指一段较短的单链RNA或DNA,它能与DNA的一条链配对提供游离的3’-OH末端以作为DNA聚合酶合成脱氧核苷酸链的起始点●DNA helicase DNA解旋酶●single-strand DNA binding protein, SSB 单链DNA结合蛋白●cooperative binding 协同结合●sliding DNA clamp DNA滑动夹●sliding clamp loader 滑动夹装载器●replisome 复制体●replicon 复制子单独复制的一个DNA单元称为一个复制子,一个复制子在一个细胞周期内仅复制一次●replicator 复制器●initiator protein 起始子蛋白●end replication problem 末端复制问题●homologous recombination 同源重组●strand invasion 链侵入●Holliday junction Holliday联结体●branch migration 分支移位●joint molecule 连接分子●synthesis-dependent strand annealing, SDSA 合成依赖性链退火●gene conversion 基因转变●conservative site-specific recombination, CSSR 保守性位点特异性重组●recombination site 重组位点●recombinase recognition sequence 重组酶识别序列●crossover region 交换区●serine recombinase 丝氨酸重组酶●tyrosine recombinase 酪氨酸重组酶●lysogenic state 溶原状态●lytic growth 裂解生长●transposon 转座子能够在没有序列相关性的情况下独立插入基因组新位点上的一段DNA序列,是存在与染色体DNA上可自主复制和位移的基本单位。
考研英语 基因鉴定及其存在的问题原文

考研英语基因鉴定及其存在的问题原文全文共3篇示例,供读者参考篇1DNA Profiling and Its Existing IssuesDNA profiling, also known as genetic fingerprinting, has revolutionized the field of forensic science and criminal investigations. This technique involves analyzing specific regions of an individual's DNA to create a unique genetic profile, which can be used to identify individuals or establish biological relationships. While DNA profiling has proven to be a powerful tool in solving crimes and exonerating the innocent, it has also raised several ethical, legal, and social concerns that warrant careful consideration.One of the primary issues surrounding DNA profiling is privacy and civil liberties. The collection and storage of genetic information raise concerns about potential misuse or unauthorized access. Critics argue that DNA databases could be exploited by governments or other entities for purposes beyond law enforcement, such as genetic discrimination or surveillance. There are also fears that DNA profiles could be used to revealsensitive information about an individual's health, ancestry, or behavioral traits, violating their right to privacy.Another concern is the accuracy and reliability of DNA evidence. While DNA profiling is considered highly accurate, there is always a possibility of errors or contamination during the collection, handling, or analysis of samples. Such errors could lead to wrongful convictions or the exoneration of guilty individuals. Additionally, the interpretation of DNA evidence can be subjective and may be influenced by cognitive biases or inadequate training of forensic experts.The issue of racial and ethnic bias in DNA profiling is also a matter of concern. Some studies have suggested that certain ethnic groups may be disproportionately represented in DNA databases due to factors such as socioeconomic status, policing practices, or historical discrimination. This could lead to increased scrutiny and potentially unjust treatment of certain communities, further exacerbating existing disparities in the criminal justice system.Another ethical consideration is the use of DNA profiling in familial searching, where law enforcement officers search DNA databases for partial matches to identify potential relatives of a suspect. While this technique has been successful in solving coldcases, it raises questions about the privacy rights of individuals who are not directly involved in a criminal investigation. Critics argue that familial searching could lead to the genetic surveillance of entire families or communities without their consent.Furthermore, the retention and destruction policies for DNA samples and profiles vary across jurisdictions, raising concerns about the potential for long-term storage and misuse of genetic information. Some argue that DNA samples and profiles should be destroyed after a certain period or upon acquittal, while others believe that retaining such information could be valuable for future investigations or exonerations.Despite these issues, DNA profiling has undoubtedly played a crucial role in solving crimes, identifying missing persons, and exonerating the wrongfully convicted. However, it is imperative that the use of this technology be accompanied by robust legal and ethical frameworks to address the concerns mentioned above.One potential solution is the implementation of strict privacy and data protection laws, ensuring that DNA profiles are used solely for lawful purposes and that individuals' genetic information is adequately safeguarded. Additionally, ongoingtraining and oversight of forensic professionals, as well as the development of standardized protocols and quality control measures, could help mitigate the risk of errors and biases in the interpretation of DNA evidence.Addressing racial and ethnic biases in DNA databases may require a multifaceted approach, including comprehensive reviews of policing practices, criminal justice reforms, and efforts to increase diversity and representation within law enforcement agencies and forensic laboratories.Regarding familial searching, some experts suggest implementing strict guidelines and oversight mechanisms to ensure that this technique is used only in exceptional circumstances and with appropriate safeguards to protect the privacy rights of individuals and their families.Lastly, clear policies and regulations surrounding the retention and destruction of DNA samples and profiles should be established, striking a balance between preserving valuable evidence for future investigations and protecting individual privacy rights.In conclusion, while DNA profiling has proven to be a powerful tool in the pursuit of justice, it is essential to address the ethical, legal, and social issues surrounding its use. Byengaging in open and informed discourse, implementing robust legal and ethical frameworks, and fostering transparency and accountability, we can harness the benefits of this technology while mitigating its potential risks and upholding the principles of fairness, privacy, and civil liberties.篇2DNA Identification and Its Existing ProblemsAs a graduate student pursuing my studies in molecular biology, I have become increasingly fascinated by the field of DNA identification and its numerous applications. From forensic investigations to paternity testing and even genealogical research, the ability to analyze and interpret an individual's genetic makeup has revolutionized various domains. However, despite its immense potential, DNA identification is not without its challenges and ethical conundrums, which warrant careful consideration.At its core, DNA identification relies on the fundamental principle that each individual's genetic code is unique, barring identical twins. This uniqueness arises from the vast number of possible combinations of nucleotide sequences that make up our DNA. By analyzing specific regions of an individual's DNA,known as Short Tandem Repeats (STRs), scientists can create a genetic profile that serves as a molecular fingerprint.The application of DNA identification in forensic science has been nothing short of groundbreaking. Traditional methods of evidence collection and analysis often fell short in cases where physical evidence was scarce or contaminated. However, the advent of DNA profiling has provided investigators with a powerful tool to link suspects to crime scenes or exonerate the wrongly accused. The ability to extract and analyze minute traces of biological material, such as hair, skin cells, or bodily fluids, has significantly improved the accuracy and reliability of forensic investigations.Another significant application of DNA identification lies in the realm of paternity testing. Historically, establishing paternal relationships relied heavily on circumstantial evidence and presumptions, leading to potential inaccuracies and emotional turmoil. DNA testing has revolutionized this process by providing a scientifically robust method for determining biological relationships. This has not only facilitated the resolution of disputes but has also played a crucial role in ensuring the well-being of children and upholding their fundamental rights.Furthermore, DNA identification has opened up new avenues in genealogical research and ancestry tracing. By comparing an individual's genetic profile to databases containing DNA samples from various populations and ethnic groups, researchers can unravel intricate family histories and shed light on migration patterns and evolutionary trajectories. This knowledge has not only satisfied personal curiosities but has also contributed to our understanding of human diversity and the complex tapestry of our shared ancestry.Despite these remarkable achievements, DNA identification is not without its fair share of challenges and controversies. One of the primary concerns revolves around the issue of privacy and the potential misuse of genetic information. As our genetic code contains a wealth of personal data, including predispositions to certain diseases and traits, there is a justified fear that this information could be exploited for discriminatory purposes, such as in employment or insurance decisions.Moreover, the collection, storage, and handling of DNA samples raise significant ethical and legal questions. While strict protocols and guidelines exist to ensure the proper management of genetic data, instances of improper handling or unauthorizedaccess can have severe consequences, ranging from breaches of privacy to potential miscarriages of justice.Another contentious issue lies in the interpretation of DNA evidence itself. While DNA profiling is generally considered highly reliable, there have been instances where factors such as contamination, degradation, or human error have led to erroneous results. Additionally, the statistical interpretation of DNA evidence can be complex, and differing methodologies may yield varying probabilities, potentially influencing legal outcomes.Furthermore, the use of DNA identification in the criminal justice system has sparked debates regarding its potential for perpetuating systemic biases. Concerns have been raised about the disproportionate representation of certain ethnic and socioeconomic groups in DNA databases, which could lead to increased scrutiny and potential profiling.Despite these challenges, the field of DNA identification continues to evolve, driven by advances in technology and a deeper understanding of genetic principles. Ongoing research efforts are focused on improving the accuracy and efficiency of DNA analysis techniques, as well as expanding the range of applications.One promising area of development is the use ofNext-Generation Sequencing (NGS) technologies, which allow for the rapid and cost-effective analysis of entire genomes. This could potentially enhance the resolution and discriminatory power of DNA profiling, facilitating more precise identifications and shedding light on complex biological relationships.Additionally, the integration of DNA identification with other cutting-edge technologies, such as machine learning and artificial intelligence, holds significant promise. These advanced computational techniques could assist in the analysis and interpretation of vast amounts of genetic data, potentially uncovering previously undetected patterns and relationships.As we navigate the intricate landscape of DNA identification, it is imperative that ethical considerations remain at the forefront. Robust governance frameworks, rigorous scientific standards, and inclusive societal dialogues are essential to ensure that the benefits of this powerful technology are maximized while mitigating potential risks and addressing legitimate concerns.In conclusion, DNA identification has revolutionized various fields, from forensics to paternity testing and genealogical research. Its ability to unlock the secrets encoded within our genetic makeup has provided invaluable insights and facilitatedthe pursuit of justice, familial connections, and self-discovery. However, as with any powerful technology, DNA identification is not without its challenges and ethical dilemmas. By addressing these concerns through ongoing research, responsible governance, and inclusive discussions, we can harness the full potential of this transformative technology while upholding the principles of privacy, fairness, and human rights.篇3DNA Identification and Its Existing ProblemsAs a student pursuing a degree in molecular biology, I can't help but be fascinated by the incredible potential of DNA identification technology. From solving criminal cases to establishing paternity and even uncovering long-lost ancestral roots, the ability to analyze and interpret an individual's unique genetic blueprint has revolutionized various fields. However, like any powerful tool, DNA identification is not without its challenges and controversies.DNA, or deoxyribonucleic acid, is the hereditary material present in nearly all living organisms, encoding the instructions for their development, functioning, and reproduction. Every person's DNA is unique, with the exception of identical twins,making it an invaluable tool for identification purposes. The process of DNA identification, also known as DNA profiling or DNA typing, involves extracting and analyzing specific regions of an individual's DNA, known as loci, to create a unique genetic profile.The applications of DNA identification are far-reaching and have had a profound impact on various aspects of society. In the realm of criminal justice, DNA evidence has played a pivotal role in solving countless cases, exonerating the innocent, and identifying perpetrators with unprecedented accuracy. By comparing DNA samples collected from crime scenes with those in forensic databases, law enforcement agencies can establish crucial links or eliminate suspects, leading to more reliable convictions or acquittals.Beyond its forensic applications, DNA identification has also been instrumental in resolving paternity disputes, enabling individuals to establish biological relationships with certainty. This technology has brought closure to many families and provided a sense of identity to those who previously lacked it. Additionally, genealogical DNA testing has gained immense popularity, allowing people to trace their ancestral roots anduncover fascinating details about their ethnic origins and family histories.While the benefits of DNA identification are undeniable, there are several ethical, legal, and social concerns that need to be addressed. One of the primary issues is the potential for misuse or abuse of genetic information. DNA profiles can reveal sensitive personal information, such as an individual's predisposition to certain diseases or inherited traits, raising privacy concerns. There is a risk that this information could be used for discriminatory purposes in areas like employment, insurance, or social interactions, leading to potential infringements on civil liberties.Another significant challenge lies in the handling and storage of DNA data. As DNA databases continue to grow, concerns arise regarding data security, potential breaches, and the mishandling of sensitive genetic information. There is a need for robust protocols and strict regulations to ensure the proper collection, storage, and access to DNA data, safeguarding individual privacy while still allowing for legitimate use by authorized entities.Furthermore, the reliability and accuracy of DNA identification techniques have been called into question incertain cases. While the technology itself is highly accurate, issues can arise due to human error, contamination of samples, or improper handling and interpretation of data. These concerns highlight the importance of adhering to stringent quality control measures and ensuring that those involved in DNA analysis are properly trained and follow established protocols.Additionally, the use of DNA identification in various contexts raises ethical and legal questions. For instance, the practice of familial searching, where law enforcement agencies search DNA databases for partial matches to identify potential relatives of a suspect, has sparked debates around privacy rights and the boundaries of acceptable investigative techniques.Moreover, the application of DNA identification in areas such as immigration enforcement and targeted surveillance of certain communities has raised concerns about discrimination and potential violations of civil liberties.As a student exploring this fascinating field, I believe it is essential to strike a delicate balance between harnessing the power of DNA identification technology and addressing the legitimate concerns surrounding its use. Comprehensive legal frameworks and robust ethical guidelines must be established togovern the collection, storage, and utilization of genetic data, ensuring that individual privacy and civil liberties are protected.Ongoing research and dialogue among scientists, policymakers, legal experts, and the public are crucial to navigate the complex issues surrounding DNA identification. Ethical considerations, such as informed consent, data security, and non-discrimination, should be at the forefront of discussions. Additionally, education and public awareness campaigns can play a vital role in fostering a better understanding of the implications and responsible use of this technology.While DNA identification has undoubtedly revolutionized various aspects of our society, it is essential to approach it with caution and a deep appreciation for its potential consequences. By addressing the existing challenges and concerns, we can harness the incredible potential of this technology while upholding the fundamental rights and dignity of individuals.In conclusion, DNA identification is a powerful tool that has transformed numerous fields, from criminal justice to genealogy. However, its widespread application and the sensitive nature of genetic information demand a vigilant approach. By actively engaging in discussions, promoting ethical practices, and continuously refining legal frameworks, we can ensure that DNAidentification technology is used responsibly and for the betterment of society as a whole.。
翻译沃森和克里克于1953年发表在《科学杂志》关于DNA双螺旋模型的论文

分子生物学作业:翻译沃森和克里克于1953年发表在《科学杂志》上的关于DNA双螺旋模型的论文Nature科学杂志Equipment,and to Dr. G. E. R. Deacon and the captain and officers of R.R.S.Discovery II for their part,in making the observations.Molecular structure of nucleic acids核酸分子结构A structure for Deoxyribose nucleic acid脱氧核糖核酸的结构We wish to suggest a structure for the salt of deoxyribose nucleic acid (D.N.A). This structure has novel features which are of considerable biological interest.我们希望去提出一种结构是刺激性的脱氧核糖核酸即DNA。
这个结构有一些新的特征对于生物学有很多重要的意义。
A structure for nucleic acid has already been proposed by Pauling and Corey2.鲍林和科瑞提出了核酸的结构。
they kindly made their manuscript available to us in advance of publication.在他们出版前,他们爽快的将对他们有用的手稿给我们。
Their model consists of three intertwined chains,with the phosphates near the fibre axis,and the bases on the outside.他们提出的模型由三个缠绕的链组成,以磷酸盐靠近纤维轴线并且盘绕在外部。
莫拉菌 基因序列的保守区域
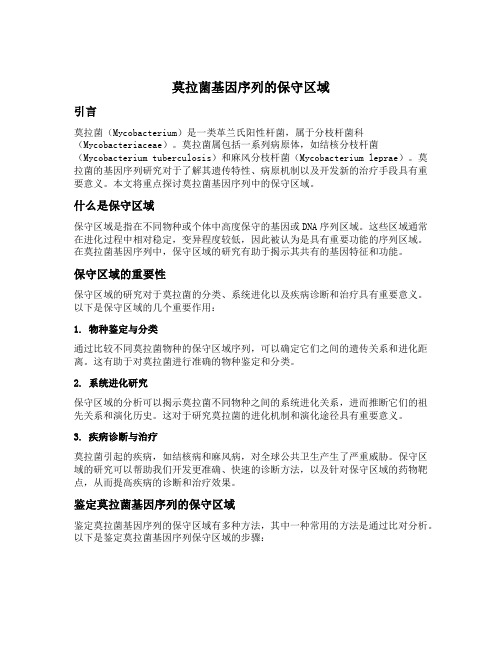
莫拉菌基因序列的保守区域引言莫拉菌(Mycobacterium)是一类革兰氏阳性杆菌,属于分枝杆菌科(Mycobacteriaceae)。
莫拉菌属包括一系列病原体,如结核分枝杆菌(Mycobacterium tuberculosis)和麻风分枝杆菌(Mycobacterium leprae)。
莫拉菌的基因序列研究对于了解其遗传特性、病原机制以及开发新的治疗手段具有重要意义。
本文将重点探讨莫拉菌基因序列中的保守区域。
什么是保守区域保守区域是指在不同物种或个体中高度保守的基因或DNA序列区域。
这些区域通常在进化过程中相对稳定,变异程度较低,因此被认为是具有重要功能的序列区域。
在莫拉菌基因序列中,保守区域的研究有助于揭示其共有的基因特征和功能。
保守区域的重要性保守区域的研究对于莫拉菌的分类、系统进化以及疾病诊断和治疗具有重要意义。
以下是保守区域的几个重要作用:1. 物种鉴定与分类通过比较不同莫拉菌物种的保守区域序列,可以确定它们之间的遗传关系和进化距离。
这有助于对莫拉菌进行准确的物种鉴定和分类。
2. 系统进化研究保守区域的分析可以揭示莫拉菌不同物种之间的系统进化关系,进而推断它们的祖先关系和演化历史。
这对于研究莫拉菌的进化机制和演化途径具有重要意义。
3. 疾病诊断与治疗莫拉菌引起的疾病,如结核病和麻风病,对全球公共卫生产生了严重威胁。
保守区域的研究可以帮助我们开发更准确、快速的诊断方法,以及针对保守区域的药物靶点,从而提高疾病的诊断和治疗效果。
鉴定莫拉菌基因序列的保守区域鉴定莫拉菌基因序列的保守区域有多种方法,其中一种常用的方法是通过比对分析。
以下是鉴定莫拉菌基因序列保守区域的步骤:1. 数据收集收集不同莫拉菌物种的基因序列数据,可以通过公共数据库如GenBank获取。
确保所收集的数据具有较高的覆盖度和质量。
2. 序列比对使用多序列比对软件如ClustalW或MAFFT对收集到的莫拉菌基因序列进行比对。
The Ethics of Gene Editing

The Ethics of Gene EditingGene editing has been a topic of debate for several years now, with scientists and ethicists divided on the ethical implications of this technology. The ability to manipulate genes and alter the genetic makeup of an organism has the potential to revolutionize medicine, agriculture, and even human evolution. However, it also raises several ethical concerns, including the possibility of creating a new class of genetically modified humans and the potential for unintended consequences.One of the primary ethical concerns surrounding gene editing is the potential for creating a new class of genetically modified humans. This could lead to a society that is divided based on genetic traits, with those who are genetically modified having advantages over those who are not. This could lead to discrimination and the creation of a genetic underclass, which is a violation of basic human rights. Additionally, there is the possibility that these genetic modifications could be passed down to future generations, leading to further genetic inequality.Another ethical concern is the potential for unintended consequences. Gene editing is a complex process that involves manipulating the genetic makeup of an organism. While scientists have made significant progress in this area, there is still much that is unknown about the long-term effects of these modifications. There is the possibility that these modifications could have unintended consequences, such as creating new diseases or causing existing ones to become more virulent.There is also the issue of consent. Gene editing has the potential to create a new class of humans, but it is unclear who would have access to this technology. If only the wealthy or privileged have access to gene editing, it could lead to further inequality and discrimination. Additionally, there is the issue of informed consent. It is unclear how much information individuals would need to make an informed decision about gene editing, and whether they would fully understand the risks and benefits of this technology.On the other hand, gene editing also has the potential to revolutionize medicine and agriculture. In medicine, gene editing could be used to cure genetic diseases, such as sicklecell anemia and cystic fibrosis. It could also be used to develop new treatments for cancer and other diseases. In agriculture, gene editing could be used to develop crops that are resistant to pests and disease, reducing the need for harmful pesticides and herbicides.In addition, gene editing could be used to address issues of social justice and equality. For example, it could be used to eliminate genetic diseases that disproportionately affect certain populations, such as sickle cell anemia in African Americans. It could also be used to address issues of food insecurity by developing crops that are more resilient to climate change and other environmental factors.In conclusion, the ethics of gene editing is a complex issue that requires careful consideration. While there are certainly potential benefits to this technology, there are also significant ethical concerns that must be addressed. The possibility of creating a new class of genetically modified humans, the potential for unintended consequences, and the issue of consent are just a few of the ethical concerns that must be addressed. Ultimately, it is up to society as a whole to decide whether the benefits of gene editing outweigh the ethical concerns.。
The Ethics of Human Genetic Editing
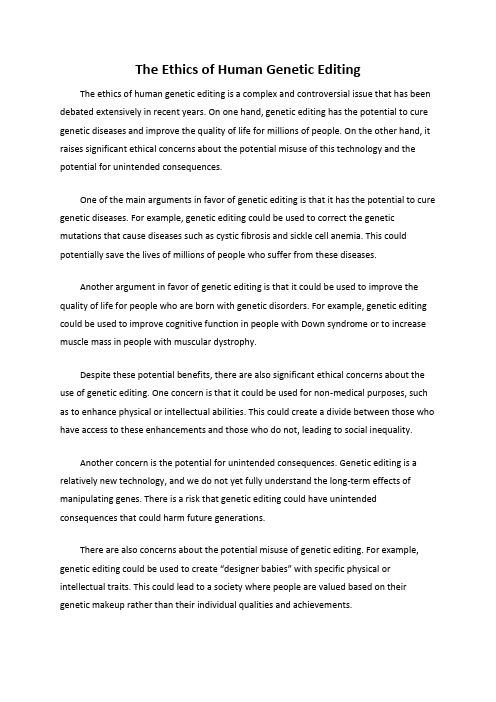
The Ethics of Human Genetic EditingThe ethics of human genetic editing is a complex and controversial issue that has been debated extensively in recent years. On one hand, genetic editing has the potential to cure genetic diseases and improve the quality of life for millions of people. On the other hand, it raises significant ethical concerns about the potential misuse of this technology and the potential for unintended consequences.One of the main arguments in favor of genetic editing is that it has the potential to cure genetic diseases. For example, genetic editing could be used to correct the genetic mutations that cause diseases such as cystic fibrosis and sickle cell anemia. This could potentially save the lives of millions of people who suffer from these diseases.Another argument in favor of genetic editing is that it could be used to improve the quality of life for people who are born with genetic disorders. For example, genetic editing could be used to improve cognitive function in people with Down syndrome or to increase muscle mass in people with muscular dystrophy.Despite these potential benefits, there are also significant ethical concerns about the use of genetic editing. One concern is that it could be used for non-medical purposes, such as to enhance physical or intellectual abilities. This could create a divide between those who have access to these enhancements and those who do not, leading to social inequality.Another concern is the potential for unintended consequences. Genetic editing is a relatively new technology, and we do not yet fully understand the long-term effects of manipulating genes. There is a risk that genetic editing could have unintended consequences that could harm future generations.There are also concerns about the potential misuse of genetic editing. For example, genetic editing could be used to create “designer babies” with specific physical or intellectual traits. This could lead to a society where people are valued based on their genetic makeup rather than their individual qualities and achievements.In conclusion, the ethics of human genetic editing is a complex issue that requires careful consideration. While genetic editing has the potential to cure genetic diseases and improve the quality of life for millions of people, it also raises significant ethical concerns about the potential misuse of this technology and the potential for unintended consequences. As we continue to develop and refine genetic editing technology, it is important to carefully consider the ethical implications of its use and to ensure that it is used in a responsible and ethical manner.。
生物专业英语试题及答案
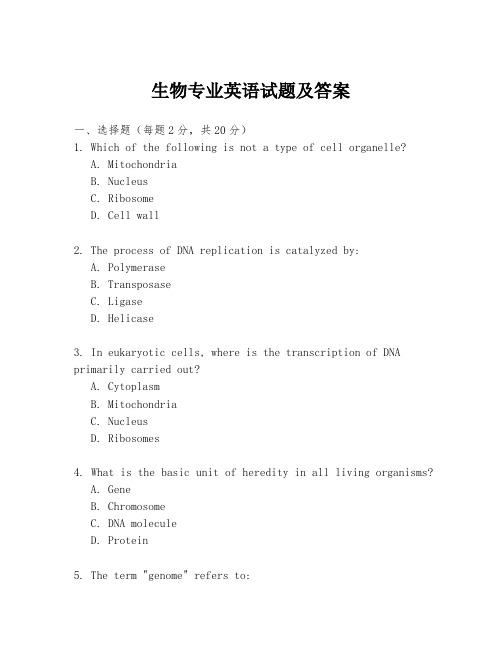
生物专业英语试题及答案一、选择题(每题2分,共20分)1. Which of the following is not a type of cell organelle?A. MitochondriaB. NucleusC. RibosomeD. Cell wall2. The process of DNA replication is catalyzed by:A. PolymeraseB. TransposaseC. LigaseD. Helicase3. In eukaryotic cells, where is the transcription of DNA primarily carried out?A. CytoplasmB. MitochondriaC. NucleusD. Ribosomes4. What is the basic unit of heredity in all living organisms?A. GeneB. ChromosomeC. DNA moleculeD. Protein5. The term "genome" refers to:A. The complete set of genes of an organismB. The entire DNA of an organismC. The sum of all the proteins in an organismD. The collection of all the cells in an organism6. Which of the following is a method of genetic engineering?A. CrossbreedingB. CloningC. CRISPR-Cas9D. Natural selection7. What is the role of tRNA in protein synthesis?A. To provide the energy for the processB. To carry specific amino acids to the ribosomeC. To serve as the template for protein synthesisD. To catalyze the formation of peptide bonds8. The Hardy-Weinberg principle states that the allele frequencies in a population will remain constant in the absence of:A. MigrationB. Genetic driftC. Natural selectionD. All of the above9. Which of the following is not a type of mutation?A. DeletionB. InsertionC. TranslocationD. Translation10. The process of photosynthesis primarily occurs in the:A. Cell wallB. CytoplasmC. ChloroplastsD. Nucleus二、填空题(每空1分,共10分)1. The chemical structure of DNA is a double ________ helix.2. The process by which a fertilized egg develops into a mature organism is called ________.3. In genetics, the term "dominant" refers to an allele that expresses its effect when ________.4. The scientific name for a species is composed of two parts: the genus name and the ________ name.5. The primary function of the Golgi apparatus is to ________, modify, and package proteins for secretion or delivery toother organelles.三、简答题(每题10分,共20分)1. Explain the difference between prokaryotic and eukaryotic cells.2. Describe the process of mitosis and its significance incell division.四、翻译题(每题15分,共30分)1. Translate the following sentence into English:"基因编辑技术,如CRISPR-Cas9,为研究和治疗遗传性疾病提供了新的可能性。
分子生物学名词解释英文
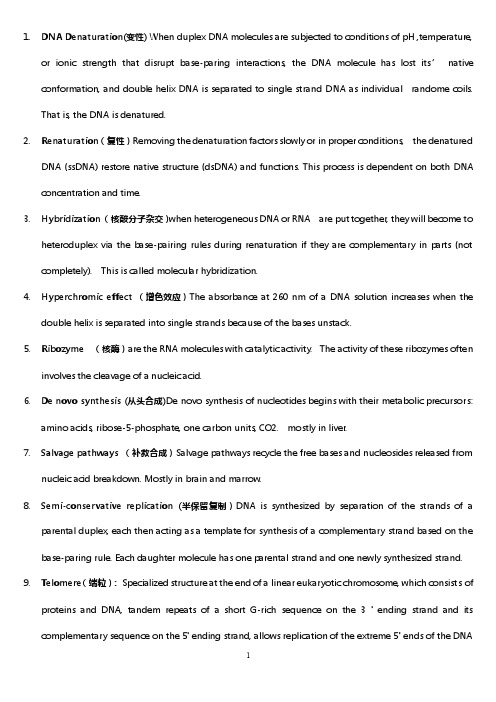
1.DNA Denaturation(变性) When duplex DNA molecules are subjected to conditions of pH ,temperature,or ionic strength that disrupt base-paring interactions, the DNA molecule has lost its’native conformation, and double helix DNA is separated to single strand DNA as individual randome coils.That is, the DNA is denatured.2.Renaturation(复性)Removing the denaturation factors slowly or in proper conditions, the denaturedDNA (ssDNA) restore native structure (dsDNA) and functions. This process is dependent on both DNA concentration and time.3.Hybridization (核酸分子杂交)when heterogeneous DNA or RNA are put together, they will become toheteroduplex via the base-pairing rules during renaturation if they are complementary in parts (not completely). This is called molecular hybridization.4.Hyperchromic effect (增色效应)The absorbance at 260 nm of a DNA solution increases when thedouble helix is separated into single strands because of the bases unstack.5.Ribozyme (核酶)are the RNA molecules with catalytic activity. The activity of these ribozymes ofteninvolves the cleavage of a nucleic acid.6.De novo synthesis (从头合成)De novo synthesis of nucleotides begins with their metabolic precursors:amino acids, ribose-5-phosphate, one carbon units, CO2. mostly in liver.7.Salvage pathways (补救合成)Salvage pathways recycle the free bases and nucleosides released fromnucleic acid breakdown. Mostly in brain and marrow.8.Semi-conservative replication (半保留复制)DNA is synthesized by separation of the strands of aparental duplex, each then acting as a template for synthesis of a complementary strand based on the base-paring rule. Each daughter molecule has one parental strand and one newly synthesized strand. 9.Telomere(端粒):Specialized structure at the end of a linear eukaryotic chromosome, which consists ofproteins and DNA, tandem repeats of a short G-rich sequence on the 3 ' ending strand and its complementary sequence on the 5' ending strand, allows replication of the extreme 5' ends of the DNAwithout loss of genetic information and maintains the stability of eukaryote chromosome.10.Telomerase(端粒酶)An RNA-containing reverse transcriptase that using the RNA as a template, addsnucleotides to the 3 ' ending strand and thus prevents progressive shortening of eukaryotic linear DNA molecules during replication.11.Reverse transcription (逆转录)Synthesis of a double-strand DNA from an RNA template.12.Reverse transcriptase (逆转录酶)A DNA polymerase that uses RNA as its template.activity: RNA-dependent DNA polymerase; RNAse H;DNA-dependent DNA polymerase13.The central dogma (中心法则)It described that the flow of genetic information is from DNA to RNA andthen to protein. According to the central dogma, DNA directs the synthesis of RNA, and RNA then directs the synthesis of proteins.14.asymmetric transcription(不对称转录)1..Transcription generally involves only short segments of aDNA molecule, and within those segments only one of the two DNA strands serves as a template.2.The template strand of different genes is not always on the same strand of DNA. That is, in anychromosome, different genes may use different strands as template.15.template strand (模板链)The DNA strand that serves as a template for transcription. (The relationshipbetween template and transcript is base paring and anti-parallel)16.non-template strand (or coding strand)(编码连)The DNA strand that opposites to the templatestrand.(Note that it has the same sequence as the synthesized RNA, except for the replacement of U with T )17.promoter i s the DNA sequence at which RNA polymerase binds to initiate transcription. It is alwayslocated on the upstream of a gene.18.Split genes (断裂基因)Split genes are those in which regions that are represented in mature mRNAs orstructural RNAs (exons) are separated by regions that are transcribed along with exons in the primary RNA products of genes, but are removed from within the primary RNA molecule during RNA processingsteps (introns).19.Exon(外显子) can be expressed in primary transcript and are the sequences that are represented inmature RNA molecules, it encompasses not only protein-coding genes but also the genes for various RNA (such as tRNAs or rRNAs)20.Intron(内含子)can be expressed and be the intervening nucleotide sequences that are removed fromthe primary transcript when it is processed into a mature RNA.21.Spliceosome(剪切体)A multicomponent complex contains proteins and snRNAs that are involved inmRNA splicing.22.Translation(翻译)The process of protein synthesis in which the genetic information present in anmRNA molecule (transcribed from DNA) determines the sequence of amino acids by the genetic codons.Translation occurs on ribosomes.23.genetic codon(密码子)The genetic code is a triplet code read continuously from a fixed starting pointin each mRNA, also called triplet. Genetic code defines the relationship between the base sequence of mRNA and the amino acid sequence of polypeptide.24.Degeneracy of code(密码子简并性)One codon encodes only one amino acid;More than 2 codons can encode the same amino acid;Most codons that encode the same amino acid have the difference in the third base of the codon.25.ORF(开放阅读框架)The nucleotideacids sequences in mRNA molecule from 5’AUG to 3’stop codon(UAA UAG UGA). It consists of a group of contiguous nonoverlapping genetic codons encoding a whole protein. Usually, it includes more than 500 genetic codons.26.Shine-Dalgarno sequence(SD)is a sequence upstream the start codon in prokaryotic mRNA that canbase pairs to a •UCCU•sequence at or very near the 3' end of 16S rRNA, thereby binding the mRNA and small ribosomal subunit by each other.27.Polyribosome(多聚核糖体)Ribosomes(10~100) are tandemly arranged on one mRNA and move in thedirection of 5’to 3’.Such a complex of one mRNA and a number ofribosomes is called polyribosome.28.signal peptide(信号肽)It is a short conservative amino terminal sequence (13~36AA) that exists ona newly synthesized secretory protein. It can direct this protein to a specific locationwithin the cell. It is subsequently cleaved away by signal peptidase; also called signal sequence and targeting sequence.29.Operon(操纵子): Bacteria have a simple general mechanism for coordinating the regulation of geneswhose products are involved in related processes: the genes are clustered on the chromosome and transcribed together. Most prokaryotic mRNAs are polycistronic. The single promoter requi red to initiate transcription of the cluster is the point where expression of all of the genes is regulated. The gene cluster, the promoter, and additional sequences that function in regulation are together called an operon. Operons that include 2 to 6 genes transcribed as a unit are common; some operons contain 20 or more genes.30.Housekeeping gene(管家基因)Genes that are expressed at a fairly consistent level throughout the cellcycle and from tissue to tissue. Usually involved in routine cellular metabolism. Often used for comparison when studying expression of other genes of interest.31.Trans-acting factors(反式作用因子):Usually considered to be proteins, that bind to the cis-actingsequences to control gene expression. The properties of different trans-acting factors:subunits of RNA polymerasebind to RNA Polymerase to stabilize the initiation complexbind to all promoters at specific sequences but not to RNA Polymerase (TFIID factor which binds to the TATA box)bind to a few promoters and are required for transcription initiation32.Cis-acting elements(顺式作用元件):DNA sequences in the vicinity of the structural portion of a genethat are required for gene expression. The properties of different cis-acting elements:contain short consensus sequencesmodules are related but not identicalnot fixed in location but usually within 200 bp upstream of the transcription start sitea single element is usually sufficient to confer a regulatory responsecan be located in a promoter or an enhancerassumed that a specific protein binds to the element and the presence of that protein is developmentally regulated33.Southern blotting:Genomic DNA (from tissues or cells) are cut by RE, separated by gelelectrophoresis and denatured in solution, then transferred to a nitrocellulose membrane for detecting specific DNA sequence by hybridization to a labeled probe. It can be used to quantitative and qualitative analyze genomic DNA, or analyze the recombinant plasmid and bacteriophage (screening DNA library).34.Northern blotting: RNA samples (from tissues or cells) are separated by gel electrophoresis anddenatured in solution, then transferred to a nitrocellulose membrane for detecting specific sequence by hybridization to a labeled probe. It can be used to detect the level of specific mRNA in some tissues (cells) and to compare the level of same gene expression in different tissues (cells) or at different development period.35.Western blotting:rotein samples are separated by PAGE electrophoresis, then electro-transferred to NCmembrane. The proteins on NC membrane hybridize with a specific antibody (1st antibody ), then the target protein binding with antibody is detected with a labeled secondary antibody (2nd antibody).Also called immunoblotting. It can be used to detect the specific protein, semi-quantify specific protein, etc.36.PBlotting technique(印迹):Transfer (blot) biological macromolecules separated in the gel and fix themto nitrocellulose/nylon membrane by diffusion, electro-transferring or vacuum absorption, then detectit.37.Nucleic acid probe(探针):DNA or RNA fragment labeled with radioisotope, biotin orfluorescent, is used to detect specific nucleic acid sequences by hybridization38.PCR: PCR is a technique for amplifying a specific DNA segment in vitro. The reaction system includeDNA template, T aq DNA pol, dNTP,short oligonucleotide primers, buffer containing Mg2+. The process including 3 steps: denature, annealing, extension39.DNA coloning(克隆):T o clone a piece of DNA, DNA is cut into fragments using restriction enzymes. Thefragments are pasted into vectors that have been cut by the same restriction enzyme to form recombinant DNA. The recombinant DNA are needed to transfer and maintain DNA in a host cell. This serial process and related technique are called DNA coloning or genetic engineering.40.Genomic DNA library(基因组DNA文库) A genomic library is a set of clones that together representsthe entire genome of a given organism. The number of clones that constitute a genomic library depends on (1) the size of the genome in question and (2) the insert size tolerated by the particular cloning vector system. For most practical purposes, the tissue source of the genomic DNA is unimportant because each cell of the body contains virtually identical DNA (with some exceptions).41.cDNA library(cDNA文库):A cDNA library represents a sample of the mRNA purified from a particularsource (either a collection of cells, a particular tissue, or an entire organism), which has been converted back to a DNA template by the use of the enzyme reverse transcriptase. It thus represents the genes that were being actively transcribed in that particular source under the physiological, developmental, or environmental conditions that existed when the mRNA was purified.42.α-complementation(α互补):Some plasmid vectors such as pUC19 carry the alpha fragment of the lacZ gene. The alpha fragment is the amino-terminus of the beta-galactosidase. Typically, the mutant E. coli host strain only carry the omega fragment, which is the carboxy-terminus of the protein. Either omegaor alpha fragment alone is nonfunctional. When the vector containing lac Z introduced into mutant E.coli, both the alpha and omega fragments are present there is an interaction and a functionally intact beta-galactosidase protein can be produced. This interaction is called alpha complementation.43.Secondary messenger(第二信使) are some small signal molecules that are generated in the cell inresponse to extracellular signals. They can activate many other downstream components. The most important second messengers are: Ca2+, cAMP, cGMP, DAG, IP3, Cer, AA and its derivatives, etc.44.Adaptor protein(衔接蛋白)A specialized protein that links protein components of the signalingpathway, These proteins tend to lack any intrinsic enzymatic activity themselves but instead mediate specific protein-protein interaction that drive the formation of protein complexes.45.Scaffolding protein(支架蛋白)A protein that assembles interacting signaling proteins intomultimolecular, it recruits downstream effectors in a pathway and enhances specificity of the signal. 46.Oncogene(癌基因)A gene whose product is involved either in transforming cells in culture or ininducing cancer in animals including virus oncogene(v-onc)and cellular-oncogene(c-onc )。
基因联合免疫细胞高级孟德尔随机化
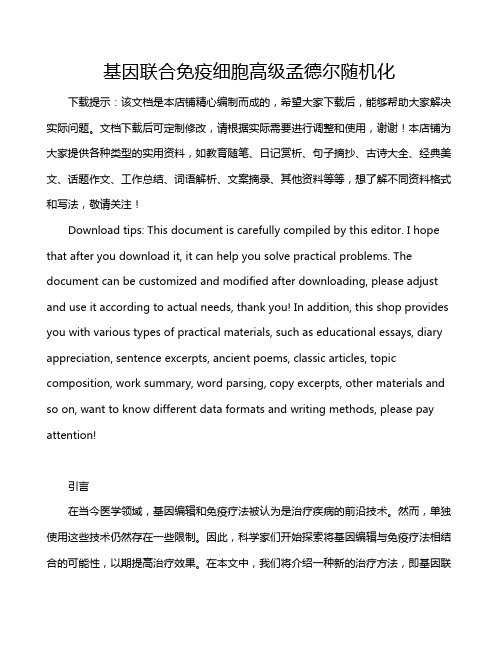
基因联合免疫细胞高级孟德尔随机化下载提示:该文档是本店铺精心编制而成的,希望大家下载后,能够帮助大家解决实际问题。
文档下载后可定制修改,请根据实际需要进行调整和使用,谢谢!本店铺为大家提供各种类型的实用资料,如教育随笔、日记赏析、句子摘抄、古诗大全、经典美文、话题作文、工作总结、词语解析、文案摘录、其他资料等等,想了解不同资料格式和写法,敬请关注!Download tips: This document is carefully compiled by this editor. I hope that after you download it, it can help you solve practical problems. The document can be customized and modified after downloading, please adjust and use it according to actual needs, thank you! In addition, this shop provides you with various types of practical materials, such as educational essays, diary appreciation, sentence excerpts, ancient poems, classic articles, topic composition, work summary, word parsing, copy excerpts, other materials and so on, want to know different data formats and writing methods, please pay attention!引言在当今医学领域,基因编辑和免疫疗法被认为是治疗疾病的前沿技术。
ADAM10基因多态性与阿尔茨海默病的相关性研究

ADAM10基因多态性与阿尔茨海默病的相关性研究作者:司君增王秀芹杨艳红邢晓玲郑立峰亓勤德朱峻岭来源:《中国现代医生》2021年第17期[关键词] ADAM10;基因多态性;阿尔茨海默病;整合素[中图分类号] R749.1 [文献标识码] A [文章编号] 1673-9701(2021)17-0001-03Study on correlation between ADAM10 gene polymorphism and Alzheimer′s diseaseSI Junzeng WANG Xiuqin YANG Yanhong XING Xiaoling ZHENG Lifeng QI Qinde ZHU JunlingDepartment of Neurology,Ji′nan City People′s Hospital,Ji′nan People′s Hospital Affiliated to Shandong First Medical University,Ji′nan 271199, China[Abstract] Objective To explore the correlation between a disintegrin and metalloproteinase 10 gene (ADAM10) rs2305421 and rs653765 polymorphisms and the genetic susceptibility of northern Chinese Han population to Alzheimer′s diseas e (AD). Methods A total of 96 AD patients(the case group) and 102 healthy people (the control group) admitted to our hospital from January 2013 to May 2020 were matched in age and gender. The ADAM10 rs2305421 and rs653765 loci were genotyped by polymerase chain reaction-restriction fragment length polymorphism (PCR-RFLP). The distribution of genotype frequency and allele frequency between the case group and the control group was compared by chi-square test. The intensity analysis of single gene nucleotide polymorphism and AD risk was expressed by odds ratio (OR) and 95% confidence interval (95%CI). Results The genotype of ADAM10 rs2305421 locus in the case group was [46(47.92)%], which was higher than that in the control group [(45 (44.12)%], with no significant difference(P>0.05). The allele frequency of ADAM10 rs2305421 in the case group was (62.50%), which was higher/lower than that in the control group(72.06%), with no significant difference(P>0.05). However, the frequency of AA genotype at ADAM10 rs653765 locus was significantly different from that of the control group(P=0.042), and the risk of AD was increased compared with GG genotype(OR=2.99, 95%CI: 1.04-8.59). In addition, the proportion of allele A in AD patients was significantly higher than that in the control group(OR=1.55, 95%CI: 1.01-2.36, P=0.043). Conclusion The polymorphism of rs653765 locus of ADAM10 gene may be related to AD in Han nationality in northern China, but it has nothing to do with rs2305421 locus.[Key words] ADAM10; Gene polymorphism; Alzheimer′s disease; Integrin阿尔茨海默病(Alzheimer′s disease,AD)是以进行性记忆力减退和认知功能障碍为主的神经退行性疾病,是痴呆最常见的类型,约占痴呆总数的60%~80%[1-2]。
aneuploidy名词解释遗传学
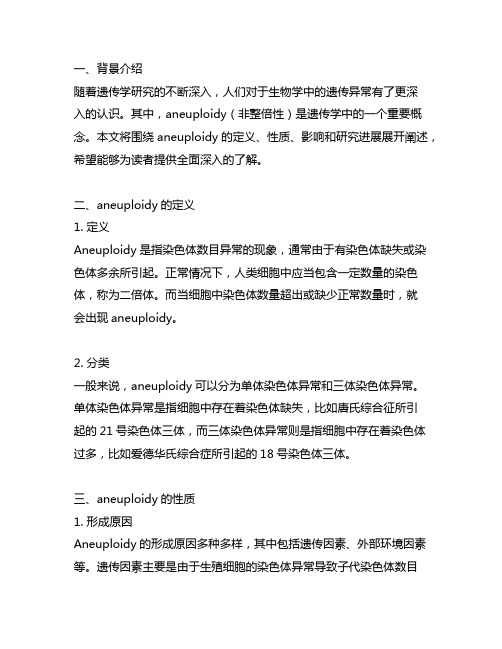
一、背景介绍随着遗传学研究的不断深入,人们对于生物学中的遗传异常有了更深入的认识。
其中,aneuploidy(非整倍性)是遗传学中的一个重要概念。
本文将围绕aneuploidy的定义、性质、影响和研究进展展开阐述,希望能够为读者提供全面深入的了解。
二、aneuploidy的定义1. 定义Aneuploidy是指染色体数目异常的现象,通常由于有染色体缺失或染色体多余所引起。
正常情况下,人类细胞中应当包含一定数量的染色体,称为二倍体。
而当细胞中染色体数量超出或缺少正常数量时,就会出现aneuploidy。
2. 分类一般来说,aneuploidy可以分为单体染色体异常和三体染色体异常。
单体染色体异常是指细胞中存在着染色体缺失,比如唐氏综合征所引起的21号染色体三体,而三体染色体异常则是指细胞中存在着染色体过多,比如爱德华氏综合症所引起的18号染色体三体。
三、aneuploidy的性质1. 形成原因Aneuploidy的形成原因多种多样,其中包括遗传因素、外部环境因素等。
遗传因素主要是由于生殖细胞的染色体异常导致子代染色体数目异常,而外部环境因素则包括放射线、化学物质等对细胞核DNA造成损伤,导致染色体异常。
2. 影响aneuploidy通常会导致个体的生理和生化功能异常,严重时甚至会导致胎儿畸形、胚胎停育等结果。
aneuploidy也可能增加罹患某些遗传性疾病的风险,比如唐氏综合征、爱德华氏综合症等。
aneuploidy对于个体的发育和健康有着重要的影响。
四、aneuploidy的研究进展1. 状态评估目前,科学家们通过观察细胞核的染色体数量、利用核型分析技术等手段来评估aneuploidy的状态。
近年来基因组学技术的不断发展也为aneuploidy的研究提供了更多的手段。
2. 研究成果研究人员通过建立动物模型、细胞培养实验等方式,不断深入探讨aneuploidy对个体发育和健康的影响机制。
在分子生物学、遗传学等领域也涌现出了大量关于aneuploidy的相关研究成果,为深入了解aneuploidy的影响机制奠定了基础。
人类基因组中长重复序列的生物学功能和疾病关联
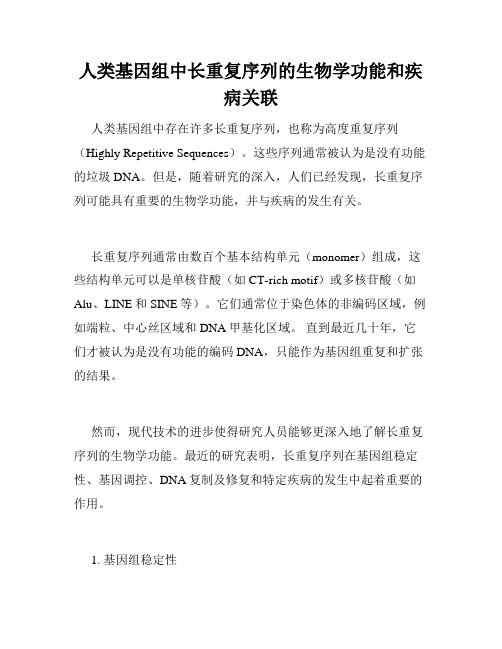
人类基因组中长重复序列的生物学功能和疾病关联人类基因组中存在许多长重复序列,也称为高度重复序列(Highly Repetitive Sequences)。
这些序列通常被认为是没有功能的垃圾DNA。
但是,随着研究的深入,人们已经发现,长重复序列可能具有重要的生物学功能,并与疾病的发生有关。
长重复序列通常由数百个基本结构单元(monomer)组成,这些结构单元可以是单核苷酸(如CT-rich motif)或多核苷酸(如Alu、LINE和SINE等)。
它们通常位于染色体的非编码区域,例如端粒、中心丝区域和DNA甲基化区域。
直到最近几十年,它们才被认为是没有功能的编码DNA,只能作为基因组重复和扩张的结果。
然而,现代技术的进步使得研究人员能够更深入地了解长重复序列的生物学功能。
最近的研究表明,长重复序列在基因组稳定性、基因调控、DNA复制及修复和特定疾病的发生中起着重要的作用。
1. 基因组稳定性长重复序列在基因组稳定性方面发挥了重要作用,它们可以作为染色体末端(端粒)的保护器,避免染色体端的衰老和退化。
此外,长重复序列还参与了染色体重组、慢性失衡、染色体断裂和恢复等基因组维护机制。
最近的研究表明,某些长重复序列的变异可能导致染色体失衡、转座和DNA断裂,从而导致肿瘤的发生。
2. 基因调控长重复序列在基因调控中也起着重要作用。
它们可以作为基因的调节区域,通过DNA甲基化和蛋白质结合调控基因的表达。
此外,长重复序列还可以影响染色质结构和组装,从而对基因调控产生影响。
最近的研究表明,某些长重复序列的变异可能导致基因调控异常,从而导致疾病的发生。
3. DNA复制和修复长重复序列在DNA复制和修复方面也起着重要的作用。
它们可以通过提供起始点、参与DNA复制和修复的基因和结构,维护DNA稳定性。
此外,长重复序列还可以通过调节DNA复制和修复的酶的活性、DNA降解和纠错机制,影响DNA的完整性和稳定性。
最近的研究表明,某些长重复序列的变异可能导致DNA复制和修复异常,导致基因突变和疾病的发生。
酵母双杂交sos招募系统原理

酵母双杂交sos招募系统原理酵母双杂交sos招募系统原理酵母双杂交sos招募系统是一种用来探究蛋白质相互作用的工具,它可以检测在生物体内发生的互作反应。
本系统是基于酵母细胞亚细胞定位的相互作用方式而设计的,通过基因的序列改造,将sos启动子与抗性序列相连接形成启动子-抗性基因转录单元,使宿主酵母细胞只在植入外源刺激信号引起物种响应后才能存活。
这种系统具有快速,经济,简便的优点,越来越多地被广泛运用于蛋白质互作及其他生化实验工作中。
酵母双杂交sos招募系统的工作原理是基于酵母的两点杂交技术,它由两个酵母双杂交质粒组成,一个是DNA结合域蛋白(域融合蛋白)-靶蛋白的质粒(活化-),另一个是招募蛋白-标签蛋白的质粒(受体-)。
质粒中含有两个重要的序列,一个是抗性基因,另一个是sos启动子。
当域融合蛋白与标签蛋白在细胞内发生相互作用时,它们分别从活化-和受体-质粒中提取sos启动子和抗性基因。
sos启动子可诱导细胞合成sos蛋白,而不合成sos蛋白的细胞死亡。
因此,只有表达具有相关特性的受体-蛋白的细胞能够存活,并表达抗性基因。
这样就可以筛选出与域融合蛋白相互作用的受体蛋白。
在这个系统中,基因操纵的可控性和标签蛋白的杂交意味着可以选择性地诱导有关物质的活性表达。
这些特点使这种技术在诊断药物,蛋白质-蛋白质相互作用的研究以及疾病诊断上有着广泛的应用。
总的来说,酵母双杂交sos招募系统是一种重要的实验方法,它可以识别和分析蛋白质之间的相互作用,对于深入研究生命科学中涉及的蛋白质结构与功能以及其在细胞间相互作用中的作用具有重要的意义。
青蛙首次基因组定序成功-英语科普-
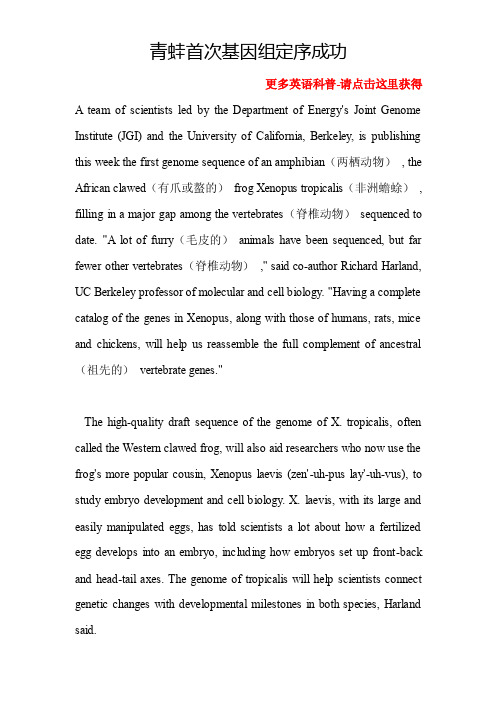
青蛙首次基因组定序成功更多英语科普-请点击这里获得A team of scientists led by the Department of Energy's Joint Genome Institute (JGI) and the University of California, Berkeley, is publishing this week the first genome sequence of an amphibian(两栖动物), the African clawed(有爪或螯的)frog Xenopus tropicalis(非洲蟾蜍), filling in a major gap among the vertebrates(脊椎动物)sequenced to date. "A lot of furry(毛皮的)animals have been sequenced, but far fewer other vertebrates(脊椎动物)," said co-author Richard Harland, UC Berkeley professor of molecular and cell biology. "Having a complete catalog of the genes in Xenopus, along with those of humans, rats, mice and chickens, will help us reassemble the full complement of ancestral (祖先的)vertebrate genes."The high-quality draft sequence of the genome of X. tropicalis, often called the Western clawed frog, will also aid researchers who now use the frog's more popular cousin, Xenopus laevis (zen'-uh-pus lay'-uh-vus), to study embryo development and cell biology. X. laevis, with its large and easily manipulated eggs, has told scientists a lot about how a fertilized egg develops into an embryo, including how embryos set up front-back and head-tail axes. The genome of tropicalis will help scientists connect genetic changes with developmental milestones in both species, Harland said."Xenopus has been among the last model organisms to be sequenced," after the mouse, chicken, nematode(线虫), zebrafish and fruit fly, he said. "It will be tremendous to have a high quality sequence of X. tropicalis upon which to build the X. laevis sequence.""The availability of the Xenopus genome also opens up the possibility of studying the effect of endocrine(内分泌的,激素的)disruptors at the molecular and genomic level," added first author Uffe Hellsten, a bioinformaticist at the JGI. These chemicals mimic frogs' own hormones, and their presence in lakes and streams may be partly responsible for the decline of frog populations worldwide."Hopefully," he added, "understanding the effects of these hormone disruptors will help us preserve frog diversity and, since these chemicals also affect humans, could have a positive effect on human health."Hellsten, Harland and 46 other scientists from 24 institutions will publish the draft genome sequence and genome-wide analysis in the April 30 issue of the journal Science.Xenopus, meaning "strange foot," is a genus of more than 20 frog species native to sub-Saharan Africa. When biologists discovered in theearly 20th century that these frogs were unusually sensitive to human chorionic gonadotropin (HCG人体绒膜促性腺激素), they were adopted widely as a low-cost pregnancy test in hospitals, primarily in the 1940s and '50s. Inject a frog with a woman's urine and, it she is pregnant, the HCG in the urine will make the frog ovulate(排卵)and produce eggs in 8-10 hours.Imported from South Africa, these frogs were kept in hospitals around the world, where scientists soon discovered their value in studying embryo development. Their large eggs also were easy to inject with chemicals; making them big spherical(球面的)test tubes, Harland said. Plus, the frogs could be induced(引诱,说服)to lay eggs at any time of year by injecting them with hormones.When the Joint Genome Institute decided to sequence a frog genome, however, the Xenopus research community recommended X. tropicalis over X. laevis because tropicalis has half the genome size. While X. tropicalis is diploid, with two copies of each gene on 10 pairs of chromosomes(染色体), the X. laevis genome has undergone duplication and could have four copies of every gene on 18 pairs of chromosomes. Sequencing X. laevis would have been not only more costly, but also harder, because of the difficulty of matching genes to the proper chromosome.Nevertheless, the high quality draft sequence will provide a "scaffold (脚手架,绞刑台)upon which to assemble the X. laevis genome," Harland said. Harland and Daniel Rokhsar, co-lead of the Xenopus genome project, UC Berkeley biology professor and JGI scientist, have applied for a National Institutes of Health grant to sequence the X. laevis genome. Harland said that, because of faster and cheaper sequencing machines, the cost would be about $1 million – about 20 times less than the cost of sequencing the X. tropicalis genome over a three-year period starting in 2002.Though the draft genome sequence has been available to scientists for several years, the new paper is the first analysis of the full genome. According to Hellsten, a comparison of regions around specific genes in the frog, chicken and human genomes shows that they are amazingly similar, indicating a high level of conservation of organization, or structure, on the chromosomes."When you look at segments of the Xenopus genome, you literally are looking at structures that are 360 million years old and were part of the genome of the last common ancestor of all birds, frogs, dinosaurs and mammals that ever roamed(漫游,流浪)the earth," said Hellsten. "Chromosome archaeology helps to understand the history of evolution,showing us how the genetic material has rearranged itself to create the present day mammalian genome and present day amphibian genome.""The rat and mouse genomes gave us the impression that genomes evolve quickly, but that turns out to be characteristic of rodents, not of all organisms," Harland said. "Instead, it seems that breaking and rearranging chromosomes are extremely rare events in evolution."The frog genome also contains genes similar to at least 1,700 genes that, in humans, are associated with disease. Thus, understanding these genes in frogs could help biologists understand how they are involved in human disease, Hellsten said.The X. tropicalis genome, which contains more than 20,000 genes –humans have about 23,000 – is of particular interest to Harland, who is part of a small community of scientists studying this frog in addition to its larger cousin, X. laevis. The frogs take up less room and have a shorter lifecycle – as little as 4 months instead of a year or more – while their smaller eggs are still relatively easy to manipulate and inject. The sequence will speed up the adoption of X. tropicalis for genetic studies in addition to developmental and cell biological studies, he said.Harland injects eggs with nucleic acids(核酸)that activate or blockthe action of specific genes or their protein products in order to discover their function."If you want to knock down multiple gene products, it's a much simpler exercise to knock them down in tropicalis, with only two copies of each gene, as opposed to knocking down or targeting gene products in laevis, where the problem is twice as complex," he said. "Now that we have a complete catalog of genes, we can also design a gene chip to look at the changes in gene expression across the whole genome, whereas previously we were restricted to the examples people had chosen to study."本文章由快乐之家/收集整理。
迟钝爱德华菌鞭毛基因克隆表达及其免疫学活性分析
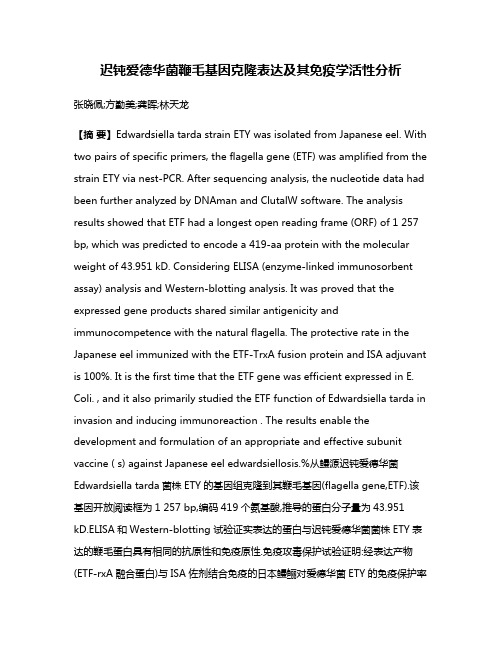
迟钝爱德华菌鞭毛基因克隆表达及其免疫学活性分析张晓佩;方勤美;龚晖;林天龙【摘要】Edwardsiella tarda strain ETY was isolated from Japanese eel. With two pairs of specific primers, the flagella gene (ETF) was amplified from the strain ETY via nest-PCR. After sequencing analysis, the nucleotide data had been further analyzed by DNAman and ClutalW software. The analysis results showed that ETF had a longest open reading frame (ORF) of 1 257 bp, which was predicted to encode a 419-aa protein with the molecular weight of 43.951 kD. Considering ELISA (enzyme-linked immunosorbent assay) analysis and Western-blotting analysis. It was proved that the expressed gene products shared similar antigenicity and immunocompetence with the natural flagella. The protective rate in the Japanese eel immunized with the ETF-TrxA fusion protein and ISA adjuvant is 100%. It is the first time that the ETF gene was efficient expressed in E. Coli. , and it also primarily studied the ETF function of Edwardsiella tarda in invasion and inducing immunoreaction . The results enable the development and formulation of an appropriate and effective subunit vaccine ( s) against Japanese eel edwardsiellosis.%从鳗源迟钝爱德华菌Edwardsiella tarda菌株ETY的基因组克隆到其鞭毛基因(flagella gene,ETF).该基因开放阅读框为1 257 bp,编码419个氨基酸,推导的蛋白分子量为43.951 kD.ELISA和Western-blotting 试验证实表达的蛋白与迟钝爱德华菌菌株ETY表达的鞭毛蛋白具有相同的抗原性和免疫原性.免疫攻毒保护试验证明:经表达产物(ETF-rxA融合蛋白)与ISA佐剂结合免疫的日本鳗鲡对爱德华菌ETY的免疫保护率可达到100%.本试验首次成功实现了ETF基因的高效表达,并初步证实了迟钝爱德华菌鞭毛可诱导产生免疫保护,为进一步研制合适的高效的迟钝爱德华菌鞭毛亚单位疫苗奠定了基础.【期刊名称】《福建农业学报》【年(卷),期】2012(027)002【总页数】6页(P113-118)【关键词】迟钝爱德华菌;鞭毛;克隆;表达【作者】张晓佩;方勤美;龚晖;林天龙【作者单位】福建省农业科学院生物技术研究所,福建福州 350003;福建省农业科学院畜牧兽医研究所,福建福州 350013;福建省农业科学院生物技术研究所,福建福州 350003;福建省农业科学院生物技术研究所,福建福州 350003;福建省农业科学院生物技术研究所,福建福州 350003【正文语种】中文【中图分类】Q959.5在鱼类的病原菌中,迟钝型爱德华菌是爱德华菌属Edwardsiella中较为常见且研究也较多的重要病原菌。
- 1、下载文档前请自行甄别文档内容的完整性,平台不提供额外的编辑、内容补充、找答案等附加服务。
- 2、"仅部分预览"的文档,不可在线预览部分如存在完整性等问题,可反馈申请退款(可完整预览的文档不适用该条件!)。
- 3、如文档侵犯您的权益,请联系客服反馈,我们会尽快为您处理(人工客服工作时间:9:00-18:30)。
BioSystems 74(2004)51–62Gene duplication and hierarchical modularity in intracellularinteraction networksJennifer Hallinan ∗ARC Centre for Bioinformatics,Institute for Molecular Biosciences,The University of Queensland,Brisbane 4072,Qld,AustraliaReceived 13June 2003;received in revised form 29January 2004;accepted 2February 2004AbstractNetworks of interactions evolve in many different domains.They tend to have topological characteristics in common,possibly due to common factors in the way the networks grow and develop.It has been recently suggested that one such common characteristic is the presence of a hierarchically modular organization.In this paper,we describe a new algorithm for the detection and quantification of hierarchical modularity,and demonstrate that the yeast protein–protein interaction network does have a hierarchically modular organization.We further show that such organization is evident in artificial networks produced by computational evolution using a gene duplication operator,but not in those developing via preferential attachment of new nodes to highly connected existing nodes.©2004Elsevier Ireland Ltd.All rights reserved.Keywords:Modularity;Hierarchical;Network;Intracellular;Yeast;Gene duplication1.IntroductionNetworks of interactions between agents arise in a wide variety of contexts,including social net-works (Newman,2001),the Internet (Albert et al.,1999;Huberman and Adamic,1999)and the world wide web (Kleinberg and Lawrence,2002;Flake et al.,2002),ecological networks (Williams and Martinez,2000),and intracellular interaction networks (Bhalla and Iyengar,1999;Uetz et al.,2000;Sole and Pastor-Santorros,2002;Jeong et al.,2000).Analysis reveals that these diverse networks frequently have topological and dynamic features in common,and it has been suggested that these commonalities arise∗Corresponding author.Tel.:+61-7-3365-7131;fax:+61-7-3365-4388.E-mail address:j.hallinan@.au (J.Hallinan).from similar processes operating during the evolution and development of the networks.Topological characteristics which are common to many naturally occurring networks include a scale-free pattern of connectivity.A scale-free net-work has no characteristic number of connections per node as does a randomly constructed network;the probability P (k )of finding a node with k connections follows a power law:P(k)∝k −γ,(1)where the scaling exponent,γ,varies with the degree distribution of the network.When the degree,k ,of the nodes of a scale-free network is plotted against the probability of occurrence of that degree,P (k ),on a log–log scale the data forms a straight line,the slope of which is γ.Interaction networks may also exhibit “small-world”properties (Watts,1999).A small-world network has a0303-2647/$–see front matter ©2004Elsevier Ireland Ltd.All rights reserved.doi:10.1016/j.biosystems.2004.02.00452J.Hallinan/BioSystems74(2004)51–62 small but significant number of short-cut connectionsbetween otherwise widely separated nodes.This or-ganization leads to characteristic topological features,including a small diameter,where diameter is definedas the longest of the shortest paths between every pairof nodes in the network.Small-world networks alsohave a large average cluster coefficient,C,comparedwith randomly connected networks with the samenumber of nodes and links.Cluster coefficient is ameasure of the extent to which the neighbors of anode are linked to each other:C=1nni=1C iN i(N i−1)/2,(2)where,n is the number of nodes in the network,C i is the number of connections between neighbors of node I,and N i is the number of neighbors of node i(Watts, 1999).Many networks further appear to be organized into a number of modules.A module is generally defined as a subnetwork of a graph,the nodes of which have more connections to other nodes within the module than to external nodes(see,for example,Ancel and Fontana, 2002;Calabretta et al.,1998;Csete and Doyle,2002; Rives and Galitski,2003).The identification of modules within a network is an NP-complete problem(Flake et al.,2002).In prac-tice,a number of algorithms have been used for the identification of modules in networks.One approach involves the analysis offlux modes(the smallest sub-networks enabling the metabolic system to operate in steady state)within the network.Many polynomial time algorithms exist forfinding the maximumflow that can be routed from a source node,to a sink node, while obeying all capacity constraints(Flake et al., 2002;Stelling et al.,2002).This approach requires a “seed node”with which to initialize the algorithm. Another approach to module detection relies upon the identification of nodes or links which lie be-tween modules.Snel et al.(2002)define such link-ers as“orthologous groups with mutually exclusive associations”,and split the network at the linkers to produce modules which appear to be biologically plausible.Similarly,Schuster et al.(2002)split the network at nodes which have more than a threshold number of links,on the contention that such highly connected hubs must be external to the modules.They used a threshold number of links of four,but point out that other values may be useful,depending upon the size of the subnets produced.Module identification can be approached as a form of cluster analysis.Hierarchical clustering algorithms are widely used,even by researchers who are not interested in the cluster tree itself.The cluster tree is simply thresholded at an arbitrary depth in order to determine thefinal clusters(Ravasz and Barabasi, 2003).Girvan and Newman(2002)produced a clus-ter tree by identifying links with high“betweenness”(Freeman,1977)and iteratively removing the links with the highest betweenness to produce a cluster tree.An interesting approach was taken by Holme et al.(2002),who combined the node-removal ap-proach with the betweenness measure to develop an algorithm in which nodes of high betweenness are iteratively removed to deconstruct the network.It has recently been suggested that in addition to a modular organization,biological networks tend to have a hierarchical structure,in which nodes are orga-nized into small modules which are,in turn,organized into larger modules,and so on(Rives and Galitski, 2003).These authors propose a method for the iden-tification of hierarchical modularity which does not require the identification of individual modules.They derive a scaling law for the connectivity of nodes in a hierarchically modular networkC(k)≈k−1where,C(k)is the cluster coefficient defined in Eq.(2).Networks whose C(k)distributionsfit this curve are held to be hierarchically modular.Ravasz et al.(2002)have identified hierarchical modularity in the metabolic networks of43different organisms. While the scaling law provides a simple means of identifying hierarchical modularity in a network,it offers no insights into the form of that modularity and,hence,does not contribute to a detailed analysis of network structure.All of the algorithms discussed above rely upon user judgement either to chose the threshold at which the network is fragmented or to validate the biologi-cal plausibility of the modules.Since the algorithms are topology-based,an objective,topology-based measure of the“goodness”of a module would be a valuable addition to the module detection algorithms. In this paper we describe a new algorithm for theJ.Hallinan/BioSystems74(2004)51–6253detection of modularity,in conjunction with an ob-jective,topology-based measure of the coherence of the modules detected.These tools are combined to produce a coherence profile which can be used to visualize the extent of hierarchical modularity of a network,compare the modular structure of networks, and identify the threshold at which a network has maximum modular coherence.The major evolutionary operators which have been implicated in the evolution of scale-free networks are the preferential attachment of new nodes to highly connected existing nodes(Albert and Barabasi,2000) and the noisy duplication of existing nodes(“gene duplication”;Pastor-Satorras et al.,2002).The rela-tive importance of these operators to the development of real networks is unclear,and probably differs from network to network.Although both of these opera-tors have been demonstrated to produce a scale-free pattern of connectivity,their effect upon the mod-ularity of the network topology has not previously been investigated.We use the coherence profile al-gorithm to examine networks evolved according to several different published algorithms and compare the modularity of the resulting networks with that of the best-characterized biological network,the protein–protein interaction network of the yeast Sac-charomyces cerevisiae.work generation2.1.The yeast protein–protein interaction network Probably the best-characterized subcellular interac-tion network is the protein–protein interaction network of the bakers’yeast,S.cerevisiae.High-throughput methods for the collection of yeast protein–protein interaction data have been developed over the last 5years(Fields and Song,1989),and large interac-tion databases exist on the Web.The data in these databases is known to be both noisy and incomplete (von Meering et al.,2002).Both false negatives(in-teractions which exist in vivo,but have not been picked up by the screens)and false positives(inter-actions which occur under the particular conditions of a yeast two-hybrid screen,but not otherwise)will occur,to an unknown extent.Further,the network which can be constructed from the yeast two-hybrid data is a static snapshot of interactions,with none of the dynamic,temporal qualities of the network in the living cell.These problems mean that considerable care must be taken to choose the most reliable data with which to work,and care must be taken not to over-interpret the results of work done using yeast two-hybrid interaction data.In an effort to use only the most reliable data available,the dataset used for these experiments is the“core”set of S.cerevisiae protein–protein in-teractions identified by Deane et al.(2002)from the Database of Interacting Proteins(DIP database; /).This data is a sub-set of the entire DIP database consisting of those in-teractions which the authors verified using two forms of computational assessment,and is,therefore,less likely to contain false positive relationships than is the DIP database as a whole,although false negatives (missing interactions)undoubtedly occur.The core dataset comprises3003interactions be-tween1788proteins(an average connectivity of1.7). It does not form a single connected component,how-ever;there are139components,of which the largest has1471proteins and2770interactions(average con-nectivity1.9).This largest connected component was used for all investigations(Fig.1).work modelsSince we are interested in the evolution of biolog-ical interaction networks,the yeast protein–protein interaction network was used as the“gold standard”network for these experiments.In order to compare the effects of different evolutionary operators,we used different operators to generate networks with the same general characteristics as the yeast network. The yeast network,and probably many other bio-logical interaction networks,have three major charac-teristics:1.A power law connectivity with a well-defined cut-off.The distribution of connectivity within the network follows a power law.A truly scale-free network obeys this distribution over a wide range of connectivities.Naturally occurring networks, however,tend to deviate from the power law at the extremes of the distribution,probably because of physical factors affecting nodes:people form54J.Hallinan /BioSystems 74(2004)51–62Fig.1.The largest connected component of the curated yeast dataset.In this diagram the circles represent proteins and the lines represent interactions between proteins.relatively fewer new relationships as they age;proteins have physical limitations to the number of binding sites they can support (Amaral et al.,2000),and so on.The yeast network displays such a “cutoff”at the tail of the distribution.2.Sparse average connectivity .Although the range of connectivities is wide,most naturally occurring networks have an average connectivity of around 2.0–2.5.The average connectivity of the core yeast network is 1.9.3.Small-world characteristics .“Small-world”net-works are characterized by a small diameter rel-ative to the number of nodes in the network,and a large cluster coefficient in comparison with that of a randomly connected network of the same size and average connectivity.We generated networks with characteristics as close as possible to the size and average connectiv-ity of the yeast protein–protein interaction network,using two published algorithms which have been demonstrated to produce scale-free networks:gene duplication (Pastor-Satorras et al.,2002);and prefer-ential attachment (Ravasz et al.,2002).In addition,we generated randomly connected networks with ap-proximately the same size and average connectivity as the yeast network.Five networks were generatedusing each algorithm.The size,average connectivity,average diameter,and average cluster coefficient of these networks are described in Table 1.2.2.1.The preferential attachment modelScale-free networks were generated using the algo-rithm described by Albert and Barabasi (2000).In this algorithm,a network grows by the addition of new nodes to an existing node k i with probability,Π,pro-portional to the connectivity k i of node i :Π(k i )=k i +1j (k j +1)(3)Albert and Barabasi’s model produces scale-freenetworks only for a subset of possible values of the parameters,p and q (see Albert and Barabasi,2000for a full analysis of the behavior of the algorithm).The network analysis program Pajek (Batagelj and Mrvar,1998)incorporates an implementation of Albert and Barabasi’s algorithm,with default parameter values which will produce a scale-free network.Starting from these defaults (m 0(initial number of nodes)=3,m (nodes added at each time step)=2,p (probability of adding a link)=0.33333,q =(probability of rewiring a link)0.333335),we iteratively modified the parame-ter values until we obtained scale-free networks which also had an average connectivity as close as possibleJ.Hallinan /BioSystems 74(2004)51–6255Table 1Characteristics of the networks used in the project NetworkNodes Edges Connectivity Diameter Cluster coefficient MeanS.D.Mean S.D.Mean S.D.Mean S.D.Mean S.D.Yeast 1471.00N/A 2770.00N/A 1.88N/A 15.00N/A 0.207800N/A Random1429.0014.382768.20 1.17 1.940.0212.200.980.0022000.001400Preferential attachment 1471.000.002892.2035.95 1.970.02 4.400.49 1.0296000.018700Gene duplication945.4057.002426.40154.162.570.0733.005.400.0000360.000045All values are averaged over five networks,except for the yeast network,which is the largest connected component of the core yeast protein–protein interaction network.to that of the yeast network.The final parameters used were m 0=2,m =1,p =0.333,q =0.334.Fig.2shows a typical example of a network grown using the preferential attachment algorithm.2.2.2.Gene duplicationGene duplication has been an important factor in the evolution of many organisms (Lynch,2002).We used a network generation algorithm based upon gene duplication,in which a gene is interpreted as a node in the network (Pastor-Satorras et al.,2002).At each time step a node is selected at random and duplicated,together with all of its links to other nodes.Links as-sociated with the new node are then added with prob-ability α,or deleted with probability δ.Fig.2.The scale-free network generated using the preferential attachment algorithm.The gene duplication model tends to generate net-works with a large number of single,unconnected nodes.The average connectivity of the largest con-nected component of the network is,therefore,con-siderably higher than the value for the network as a whole.In addition,the highly stochastic nature of the algorithm means that the average connectivity of the network varies considerably from run to run of the algorithm,particularly when generating a relatively small network.Table 2summarizes the results of 100runs of the algorithm with the parameters described in Pastor-Satorras et al.(2002).The average connectivity of the largest connected component in a gene duplication network is depen-dant upon the link deletion parameter δ.It proved56J.Hallinan /BioSystems 74(2004)51–62Table 2Node and link statistics for whole network and largest connected component of the same network,averaged over 100runs of the gene duplication algorithm with δ=0.562,N =2000and k =2.5Nodes Links Average connectivity MeanS.D.Mean S.D.Mean S.D.Whole net 200005075.82020.63 2.5379 1.0103Largest CC490.0162.24797.2162.239.51661.1643impossible to find a value of δwhich would produce a giant component corresponding to that of the yeast network.A very high value of δyields a highly frag-mented network with no single large connected com-ponent,while lower δproduced larger components with connectivity somewhat higher than that of the yeast network.A value of δ(0.75)which would result in a giant component with average connectivity of around 2.5(within the range of average connectivities reported for real networks)was selected empirically.In order to generate a largest connected component of a useful size,networks of 10,000nodes were gener-ated and the largest connected component extracted.Fig.3shows the largest connected component of a typical network generated using the gene duplication algorithm.2.2.3.Random networksControl networks were generated with the appropri-ate numbers of nodes and links,connected atrandomFig.3.A network generated using the gene duplication algorithm.There are 943nodes and 2500links.so that the resulting network has approximately the same average connectivity as the yeast network.These random networks do not have scale-free connectivity,and would not be expected to display any signifi-cant modularity.An example of a random network is shown in Fig.4.3.Quantifying hierarchical modularity 3.1.Iterative vector diffusionThe iterative vector diffusion algorithm operates in the context of a graph G consisting of a vertex set V(G)={v 1...v n }and an edge set E(G)={e 1...e m }where each edge consists of two vertices.The algorithm is initialized by assigning to each vertex a binary vector of length n ,initialized tov i,j =0,i =j1,i =j where,i is an index into the vector and j is the unique number assigned to a given node.This generates an initial set of n orthogonal vectors.The algorithm proceeds iteratively.At each itera-tion an edge from the network is selected at random and the vectors associated with each of its nodes are moved towards each other by adding a small amount,δ,to each element of the vector.This vector diffusion process is iterated until a stopping criterion is met.We chose to compute a maximum number of iterations as the stopping criterion.This number,n ,is dependant upon both the number of connections in the network,c ,and the size of δ,such thatn =c × αδ,where,αis the average amount by which a vector is changed in the course of the run.A value for αofJ.Hallinan/BioSystems74(2004)51–6257Fig.4.A random network with1432nodes and2770edges.0.1was selected empirically in trials on artificially generated networks.At the end of the vector diffusion process the vec-tors,initially mutually orthogonal,are clustered in n-dimensional space.To reduce the dimensionality of the data set,the vectors are then subjected to hi-erarchical clustering using the hierarchical clustering algorithm implemented by Eisen et al.(1998).This algorithm uses the Pearson correlation coefficient as a distance metric.It calculates the distance matrix for all members of the input set of vectors and then uses an agglomerative hierarchical algorithm to create a hierarchical cluster tree,in which the two closest items in the set are joined by a node of the tree,and the two items replaced by a single item representing the new node.The process iterates until only one item remains.3.2.Cluster thresholdingThe output of the cluster algorithm is a binary tree, with a single root node giving rise to two offspring nodes,each of which give rise to two child nodes of their own,and so on.The tree can,therefore,be thresholded at various levels(two parents,four par-ents,eight parents,etc.;see Fig.5)and the modularity of the network at each level can be examined.3.3.Modular coherenceThe problem of identifying modules in a network is essentially an unsupervised cluster analysis task. Nodes are identified as belonging to a given mod-ule,or cluster,on the basis of their closeness to other nodes as assessed using an appropriate metric.There are many cluster analysis algorithms,several of which have been applied to the module detection task,as dis-cussed above.Most clustering algorithms,however, will identify“clusters”in any dataset,whether or not these have any correspondence to real groupings inthe Fig.5.Thresholding a cluster tree.(a)Tree thresholded at parent level2produces two clusters,(b)the same tree thresholded at parent level3has four clusters.58J.Hallinan/BioSystems74(2004)51–62 dataset.In order to validate the output of a clusteringalgorithm,practitioners often examine measures suchas inter-and intra-cluster variance.Such measures arenot easily applied to nodes on a graph.We propose ameasure of modular coherence,which measures therelative proportions of inter-and intra-module linksand assigns a value in the range−1(no coherence)to+1(a fully connected,stand-alone subgraph).The coherence,χ,of a previously identified modulecan be defined asχ=2k in(n−1)−1nnj=1k jik jo+k ji(4)where,k i is the total number of edges between nodesin the module,n is the number of nodes in the net-work,k ji is the number of edges between node j andother nodes within the module,and k jo is the numberof edges between node j and other nodes outside themodule.Thefirst term in this equation is simply the propor-tion of possible links between the nodes comprisingthe module which actually exist;a measure of the con-nectivity within the module.The second term is theaverage proportion of edges per node which are inter-nal to the module.A highly connected node with fewexternal edges will,therefore,have a lower value of χthan a highly connected node with many external edges.χwill have a value in the range(−1,+1).The concept of modularity in a network leads nat-urally to the question of scale.At what scale shouldmodularity be sought?It is important that any charac-teristic scale for modularity in the network arise fromthe data,rather than being imposed by the investiga-tor,since the appropriate scale cannot be determined apriori.This consideration has led to the concept of hi-erarchical modularity:the idea that network modulescan occur at a range of scales,with modules higherup the hierarchy divided into smaller modules,and soon.Holme et al.(2002)consider a fundamental ques-tion in biological network analysis to be:“what thehierarchical organization of subnetworks looks like”.Rather than making an a priori decision about thescale at which network modularity should be analyzed,we propose an approach which provides an overviewof the degree of modularity present in a given networkat every possible scale of modularity.The resultinggraph facilitates visual inspection of the modularity of the network over all possible scales,and permits the selection of a specific“characteristic scale”of the network for further analysis,if required.We call this approach a“coherence profile”.At each level in the hierarchy the number of mod-ules and the average modular coherence of the network was computed.Average coherence was then plotted against threshold level to produce the coherence pro-file summarizing the hierarchical modularity of the network.4.ResultsThe coherence profile for the yeast network is shown in Fig.6.It is immediately apparent that the yeast network has significant positive modular coherence over most of the range of thresholds.At low threshold values, corresponding to a partitioning of the network into a small number of relatively large modules,average coherence dips below0,indicating that the modules have more external than internal connectivity.This is because a clustering algorithm is part of the module identification algorithm.Any clustering algorithm will identify“clusters”in whatever data it is given;whether or not they reflect real modules in the biological net-work.Only when the measured modular coherence is positive can confidence be placed in the biological re-ality of the modules.The spurious nature of the results at high threshold levels is also indicated by the sudden increase in standard deviation at the point where mod-ular coherence drops below zero.These modules are illusory.The yeast network has approximately equal coherence over most thresholds,indicating that the network has a strongly hierarchically modular organi-zation.In contrast to the yeast network,the random network (Fig.7)shows negative coherence over most of its range.Although the clustering algorithm is still identify-ing modules,as expected,they have no coherence, have more external than internal edges,and do notfit the definition of a module given earlier.At the higher threshold levels,coherence rises slightly above zero. Inspection of the clustered network reveals that the modules detected at the extreme of the graph are an artefact of the unequal lengths of the branches of theJ.Hallinan /BioSystems 74(2004)51–6259-0.8-0.6-0.4-0.200.20.40.6ThresholdFig.6.Coherence profile for the core S.cerevisiae protein–protein interaction network.The data is the mean of 100runs of the algorithm on the same network.Dashed lines represent ±1standarddeviation.-0.4-0.3-0.2-0.100.10.20.30.4ThresholdFig.7.Coherence profile for random networks with approximately the same number of nodes and edges as the yeast network.The data is the mean of 100runs of the algorithm for each of five randomly generated networks.Dashed lines represent ±1standard deviation.cluster tree.At the extremes of the tree,there tends to be one large cluster and a number of very small (one or two node)clusters.Within the large cluster most edges will lie between nodes in the same cluster.The number of tiny clusters,most of whose edges connect to nodes external to the cluster,is too small to drive the mean coherence below zero.The random network,therefore,shows no evidence of hierarchical modular-ity,or,indeed,of significant modularity at any level.The fact that the yeast network displays hierarchical modularity,while the random network does not,pro-vides a benchmark against which to assess the biolog-ical plausibility of the network evolution algorithms discussed in the introduction.The coherence profiles for the preferential attach-ment and gene duplication networks are shown in Figs.8and 9,respectively.The preferential attachment algorithm has been shown to produce scale-free networks (Albert and Barabasi,2000).Fig.8shows,however,that these networks exhibit no sign of modularity at any level of the hierarchy.In contrast,the net-works generated by the gene duplication algo-rithm have a coherence profile very similar to that of the yeast protein–protein interaction net-work,with significant modular coherence present at almost every level of the hierarchy.It appears that gene duplication is more likely to produce a。