Electrophysiological correlates of recollecting faces of known and unknown individuals
期刊electrophoresis简介
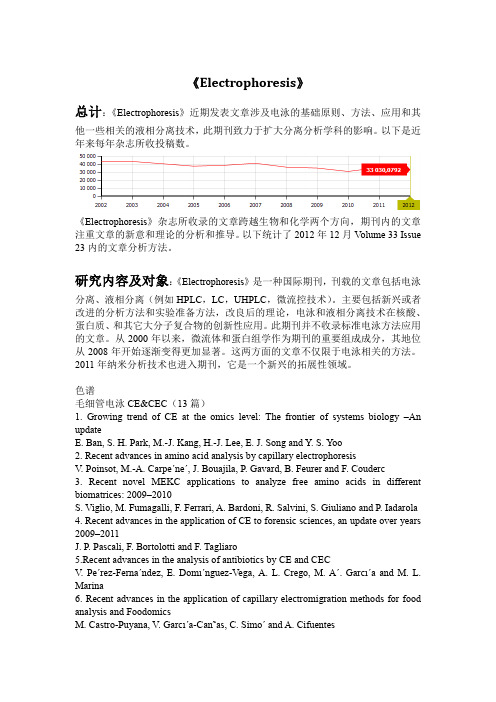
《Electrophoresis》总计:《Electrophoresis》近期发表文章涉及电泳的基础原则、方法、应用和其他一些相关的液相分离技术,此期刊致力于扩大分离分析学科的影响。
以下是近年来每年杂志所收投稿数。
《Electrophoresis》杂志所收录的文章跨越生物和化学两个方向,期刊内的文章注重文章的新意和理论的分析和推导。
以下统计了2012年12月V olume 33 Issue 23内的文章分析方法。
研究内容及对象:《Electrophoresis》是一种国际期刊,刊载的文章包括电泳分离、液相分离(例如HPLC,LC,UHPLC,微流控技术)。
主要包括新兴或者改进的分析方法和实验准备方法,改良后的理论,电泳和液相分离技术在核酸、蛋白质、和其它大分子复合物的创新性应用。
此期刊并不收录标准电泳方法应用的文章。
从2000年以来,微流体和蛋白组学作为期刊的重要组成成分,其地位从2008年开始逐渐变得更加显著。
这两方面的文章不仅限于电泳相关的方法。
2011年纳米分析技术也进入期刊,它是一个新兴的拓展性领域。
色谱毛细管电泳CE&CEC(13篇)1. Growing trend of CE at the omics level: The frontier of systems biology –An updateE. Ban, S. H. Park, M.-J. Kang, H.-J. Lee, E. J. Song and Y. S. Y oo2. Recent advances in amino acid analysis by capillary electrophoresisV. Poinsot, M.-A. Carpe´ne´, J. Bouajila, P. Gavard, B. Feurer and F. Couderc3. Recent novel MEKC applications to analyze free amino acids in differentbiomatrices: 2009–2010S. Viglio, M. Fumagalli, F. Ferrari, A. Bardoni, R. Salvini, S. Giulianoand P. Iadarola 4. Recent advances in the application of CE to forensic sciences, an update overyears 2009–2011J. P. Pascali, F. Bortolotti and F. Tagliaro5.Recent advances in the analysis of antibiotics by CE and CECV. Pe´rez-Ferna´ndez, E. Domı´nguez-V ega, A. L. Crego, M. A´.Garcı´aand M. L. Marina6. Recent advances in the application of capillary electromigration methods forfood analysis and FoodomicsM. Castro-Puyana, V. Garcı´a-Can˜as, C. Simo´ and A. Cifuentes7. CE and CEC analysis of phytochemicals in herbal medicinesX.-j. Chen, J. Zhao, Y.-t.Wang, L.-q.Huang and S.-P. Li8. Capillary electrophoresis of natural products: Highlights of the last five years(2006–2010)H. R. Rabanes, A. M. Guidote Jr. and J. P. Quirino9. CE of inorganic species – A review of methodological advancements over2009–2010P. Kuba´nˇ and A. R. Timerbaev10. Recent developments and applications of EMMA in enzymaticand derivatization reactionsX. Hai, B.-f. Y ang and A. V an Schepdael11. Recent approaches in sensitive enantioseparations by CEL. Sa´nchez-Herna´ndez, M. Castro-Puyana, M. L. Marina and A. L. Crego12. Developments in coupled solid-phase extraction–capillary electrophoresis2009–2011R. Ramautar, G. J. de Jong and G. W. Somsen13. Organic monoliths for hydrophilic interaction electrochromatography/chromat ography and immunoaffinity chromatographyD. N. Gunasena and Z. El Rassi液相分离Liquid-phase-based separation(1篇)1. Liquid-phase-based separation systems for depletion, prefractionation andenrichment of proteins in biological fluids and matrices for in-depth proteomicsanalysis – An update covering the period 2008–2011S. Selvaraju and Z. El Rassi微流体和微型化Microfluidic&Miniaturisation(2篇)1. Surface modification for PDMS-based microfluidic devicesJ. Zhou, D. A. Khodakov, A. V. Ellis and N. H. V oelcker2. Recent advances in miniaturisation –The role of microchip electrophoresisin clinical analysisF. Shang, E. Guihen and J. D. Glennon基本资料期刊名ELECTROPHORESIS ELECTROPHORESIS出版周期Semimonthly出版ISSN0173-0835通讯方式WILEY-V C H VERLAG GMBH, PO BOX 10 11 61, WEINHEIM, GERMANY, D-69451 期刊主页网址/journal/10.1002/(ISSN)1522-2683在线投稿网址/elpho/其他相关链接Science Citation IndexScience Citation Index Expanded Current Contents - Life Sciences BIOSIS Previews虫友提供资料( 8 人参与,3141 人阅读)偏重的研究方向色谱分析(1) 分析化学(1) 芯片技术(1)凝胶电泳(1) CE &CEC(1) CE(1) CEC(1) CE and CEC(1) Microfluidics andMiniaturization(1) Proteomics and 2-DE(1) 化学科学(1)投稿录用比例0%审稿速度平均2.5个月的审稿周期期刊“小木虫投稿价值”历年趋势图(投稿价值趋势图供投稿时选择参考。
电化学方法与原理 英文
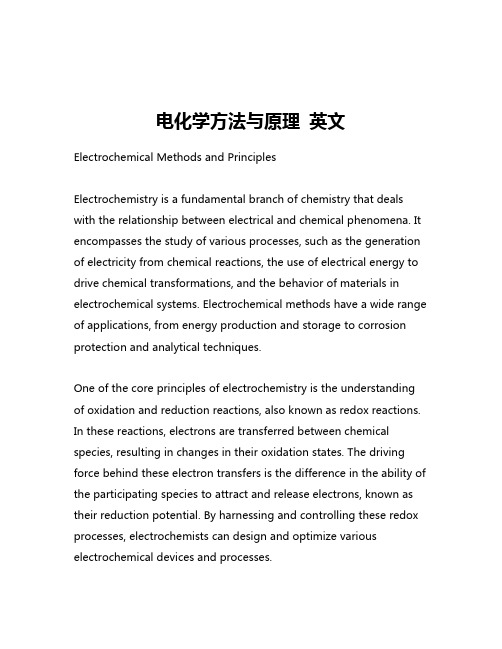
电化学方法与原理英文Electrochemical Methods and PrinciplesElectrochemistry is a fundamental branch of chemistry that deals with the relationship between electrical and chemical phenomena. It encompasses the study of various processes, such as the generation of electricity from chemical reactions, the use of electrical energy to drive chemical transformations, and the behavior of materials in electrochemical systems. Electrochemical methods have a wide range of applications, from energy production and storage to corrosion protection and analytical techniques.One of the core principles of electrochemistry is the understanding of oxidation and reduction reactions, also known as redox reactions. In these reactions, electrons are transferred between chemical species, resulting in changes in their oxidation states. The driving force behind these electron transfers is the difference in the ability of the participating species to attract and release electrons, known as their reduction potential. By harnessing and controlling these redox processes, electrochemists can design and optimize various electrochemical devices and processes.Electrochemical cells are the fundamental building blocks of electrochemical systems. These cells consist of two electrodes, an anode and a cathode, immersed in an electrolyte solution. The anode is where oxidation occurs, and the cathode is where reduction takes place. The electrolyte provides the necessary ionic conduction between the two electrodes, allowing the flow of ions and the completion of the overall electrochemical reaction.One of the most widely recognized applications of electrochemistry is energy conversion and storage. Electrochemical cells, such as batteries and fuel cells, convert the chemical energy stored in fuels or reactants directly into electrical energy. Batteries, for example, use the principle of redox reactions to generate a flow of electrons, which can then be used to power various electronic devices. Fuel cells, on the other hand, generate electricity by combining fuel (such as hydrogen) and an oxidant (such as oxygen) in an electrochemical reaction.In addition to energy applications, electrochemical methods are also used in a variety of analytical techniques. Electroanalytical methods, such as potentiometry, voltammetry, and electrochemical sensors, utilize the principles of electrochemistry to detect and quantify the presence of specific chemical species in a sample. These techniques are widely used in fields like environmental monitoring, healthcare, and chemical analysis.Corrosion is another area where electrochemistry plays a crucial role. Corrosion is an electrochemical process that involves the deterioration of materials, usually metals, due to their interaction with the surrounding environment. Understanding the electrochemical principles underlying corrosion enables the development of effective strategies for corrosion prevention and mitigation, such as the use of protective coatings, cathodic protection, and the selection of corrosion-resistant materials.Electrochemistry also finds applications in the synthesis and processing of materials. Electrochemical techniques, such as electroplating and electrodeposition, are used to deposit thin filmsor coatings of various materials onto a substrate. These processes are employed in the production of electronic components, decorative finishes, and protective coatings.The field of electrochemistry is constantly evolving, with new developments and applications emerging as our understanding of the underlying principles expands. Researchers continue to explore innovative electrochemical technologies, such as energy storage systems, fuel cells, and electrochemical sensors, to address pressing global challenges related to energy, the environment, and healthcare.In conclusion, electrochemical methods and principles arefundamental to a wide range of scientific and technological fields. From energy conversion and storage to analytical techniques and material processing, the principles of electrochemistry underpin numerous important processes that shape our modern society. As we continue to push the boundaries of scientific knowledge, the importance of electrochemistry will only grow, making it a crucial area of study for scientists and engineers alike.。
利用电子自旋共振(ESR)技术研究啤酒原辅料自由基及其对麦汁自由基的影响
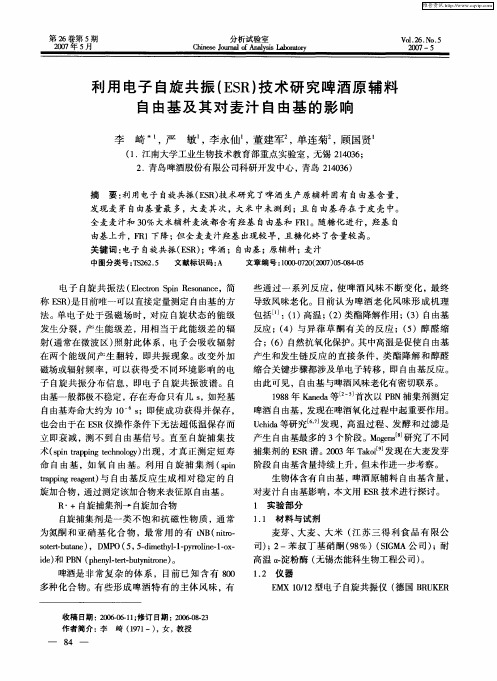
合 ; 6 然抗氧化保护。 中高温是促使 自由基 ()自 其
产生 和发生 链 反 应 的直 接 条件 ,类 酯 降 解 和 醇 醛
缩合关键步骤都涉及单电子转移 , 自由基反应。 即
由此 可见 ,自由基 与啤酒 风 味老化有 密切联 系。 18 98年 K nd aea等 首 次 以 P N捕集 剂 测 定 2 B
麦芽 、 麦 、大 米 ( 苏 三 得 利食 品 有 限公 大 江
司 ) 2一苯 叔 丁基 硝 酮 (8 ; 9 %)( IM SG A公 司 ) ;耐
高温 a淀 粉酶 ( 锡杰 能科生 物工程 公 司) 一 无 。 12 仪器 . E X 1/2型 电子 自旋 共振 仪 ( 国 B U E M 01 德 R KR
对麦 汁 自由基影 响 , 本文 用 E R技术进 行பைடு நூலகம் 讨 。 S 1 实验部分
1 1 材 料与试 剂 .
立即衰减 , 测不 到 自由基信号。 直至 自旋捕集 技
术 (p ap gt ho g ) 现 , 真 正 测 定 短 寿 si t p i cnl y 出 nr n e o 才
命 自由基 ,如 氧 自由基 。利 用 自旋 捕 集 剂 (p si n tpi aet与 自 由基 反 应 生 成 相 对 稳 定 的 自 r pn r gn) a ge 旋加合 物 , 通过 测定该 加合物 来表 征原 自由基 。 R・ +自旋捕 集剂一 自旋 加合物 自旋 捕集 剂 是 一 类不 饱 和 抗 磁 性 物 质 , 常 通
维普资讯
第2 6卷第 5期 2O 年 5月 O7
分析试验室
Ch eeJu a f ayi L b rtr i s or lo Anlss a oaoy n n
电化学催化的英文
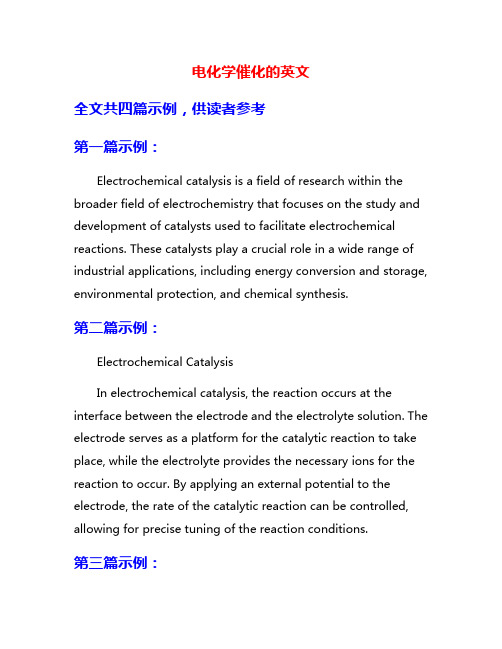
电化学催化的英文全文共四篇示例,供读者参考第一篇示例:Electrochemical catalysis is a field of research within the broader field of electrochemistry that focuses on the study and development of catalysts used to facilitate electrochemical reactions. These catalysts play a crucial role in a wide range of industrial applications, including energy conversion and storage, environmental protection, and chemical synthesis.第二篇示例:Electrochemical CatalysisIn electrochemical catalysis, the reaction occurs at the interface between the electrode and the electrolyte solution. The electrode serves as a platform for the catalytic reaction to take place, while the electrolyte provides the necessary ions for the reaction to occur. By applying an external potential to the electrode, the rate of the catalytic reaction can be controlled, allowing for precise tuning of the reaction conditions.第三篇示例:Electrochemical catalysis is a key area of research in the field of chemistry, with immense potential for applications in various industries such as energy storage, fuel cells, water purification, and organic synthesis. This field involves the use of electrochemical processes to accelerate chemical reactions by providing an alternative pathway for the reaction to occur with a lower activation energy.第四篇示例:One of the key benefits of electrochemical catalysis is its ability to control reaction kinetics and selectivity through precise tuning of electrode potentials and reaction conditions. By applying an external voltage to the electrode, researchers can modulate the energy barriers for specific chemical reactions, thereby enhancing the desired reaction pathways while suppressing unwanted side reactions. This level of control is particularly important for complex multi-step reactions, where traditional catalytic approaches may struggle to achieve high selectivity and efficiency.。
文献检索试题和答案

《大学生信息检索概论》模拟试题一、填空题1、文献的级次分为零次文献、一次文献、二次文献、三次文献2、《中图法》有五个基本部类,分别是马克思主义、列宁主义、毛泽东思想_、哲学;社会科学;自然科学和综合性图书,在此基础上又划分为_22_个大类。
3、按内容可将计算机检索系统的数据库类型分为:文献书目型数据库、事实型数据库、数值型数据库和全文型数据库。
4、我国标准可分为国家标准、部标准和企业标准三大类。
5、在实际检索中,文献的检索方法主要有:直查法、追溯法、工具法和综合法。
6、国际标准化组织简称:ISO 、本标准每5 年修订一次二、选择题1、如果需要检索某位作者的文献被引用的情况,应该使用(C )检索。
A.分类索引B.作者索引C.引文索引D.主题索引2、利用图书馆的据库检索期刊论文时,可供选择的中文数据库是(D )。
A.超星数字图书馆B.万方学位论文C.国研网D.维普科技期刊 E.高校财经库3、如果检索有关多媒体网络传播方面的文献,检索式为(A D)。
A.多媒体and 网络传播B.多媒体+网络传播C.多媒体or 网络传播D.多媒体*网络传播4、如果对某个课题进行主题检索时,可选择的检索字段有( A D E )。
A.关键词B.作者C.刊名D.题名E.文摘5、二次文献又称检索工具,包括:(A CD )。
A.书目B.百科C.索引D.文摘E.统计数据三、名词解释题1、文献用文字、图形、符号、声频、视频等技术手段记录人类知识的一种载体,或理解为固化在一定物质载体上的知识。
也可以理解为古今一切社会史料的总称。
2、体系分类语言体系语言是以科学分类为基础,运用概念的划分与概括的逻辑方法,形成一个概念等级体系,按知识门类的逻辑次序,按照从总到分,从一般到具体,从低级到高级,从简单到复杂的原则进行概念的综分,层层划分,累累隶属,逐步展开而形成的一个等级体系。
3、引文语言引文语言是根据文献所附参考或引用文献的特征进行检索的语言。
电催化析氢的英文
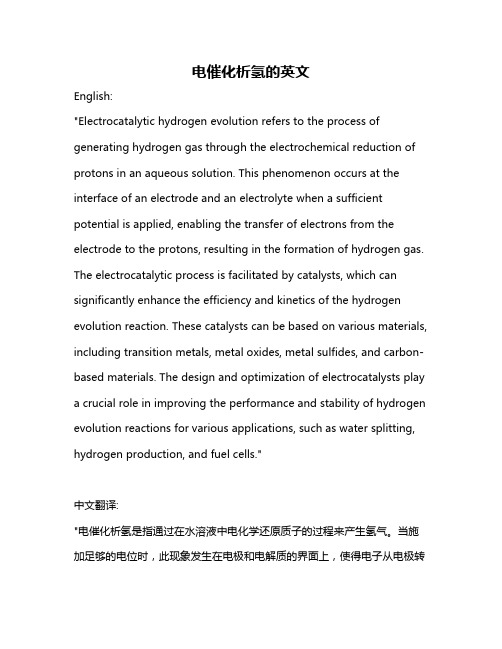
电催化析氢的英文English:"Electrocatalytic hydrogen evolution refers to the process of generating hydrogen gas through the electrochemical reduction of protons in an aqueous solution. This phenomenon occurs at the interface of an electrode and an electrolyte when a sufficient potential is applied, enabling the transfer of electrons from the electrode to the protons, resulting in the formation of hydrogen gas. The electrocatalytic process is facilitated by catalysts, which can significantly enhance the efficiency and kinetics of the hydrogen evolution reaction. These catalysts can be based on various materials, including transition metals, metal oxides, metal sulfides, and carbon-based materials. The design and optimization of electrocatalysts play a crucial role in improving the performance and stability of hydrogen evolution reactions for various applications, such as water splitting, hydrogen production, and fuel cells."中文翻译:"电催化析氢是指通过在水溶液中电化学还原质子的过程来产生氢气。
电催化反应的英文
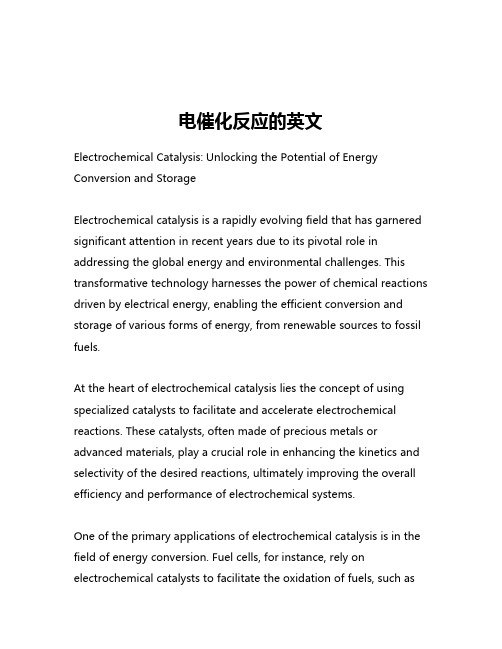
电催化反应的英文Electrochemical Catalysis: Unlocking the Potential of Energy Conversion and StorageElectrochemical catalysis is a rapidly evolving field that has garnered significant attention in recent years due to its pivotal role in addressing the global energy and environmental challenges. This transformative technology harnesses the power of chemical reactions driven by electrical energy, enabling the efficient conversion and storage of various forms of energy, from renewable sources to fossil fuels.At the heart of electrochemical catalysis lies the concept of using specialized catalysts to facilitate and accelerate electrochemical reactions. These catalysts, often made of precious metals or advanced materials, play a crucial role in enhancing the kinetics and selectivity of the desired reactions, ultimately improving the overall efficiency and performance of electrochemical systems.One of the primary applications of electrochemical catalysis is in the field of energy conversion. Fuel cells, for instance, rely on electrochemical catalysts to facilitate the oxidation of fuels, such ashydrogen or methanol, and the reduction of oxygen, generating electricity in a clean and efficient manner. The development of highly active and durable electrocatalysts has been a driving force behind the advancement of fuel cell technology, enabling the widespread adoption of these clean energy devices in various sectors, including transportation, stationary power generation, and portable electronics.Similarly, electrochemical catalysis plays a pivotal role in the storage and conversion of energy from renewable sources. In the case of water electrolysis, catalysts are employed to split water molecules into hydrogen and oxygen, allowing for the storage of energy in the form of hydrogen, which can then be used as a clean fuel or converted back into electricity through fuel cells. This process is particularly important for the integration of renewable energy sources, such as solar and wind, into the energy grid, as it provides a means to store excess energy generated during periods of high production.Moreover, electrochemical catalysis is essential in the developmentof advanced energy storage technologies, such as rechargeable batteries and metal-air batteries. Catalysts are used to enhance the efficiency and durability of the electrochemical reactions that occur during charging and discharging, enabling the storage and retrieval of energy with improved performance and safety.Beyond energy applications, electrochemical catalysis has also found important uses in the fields of environmental remediation and chemical synthesis. In the former, catalysts are employed to facilitate the electrochemical treatment of wastewater, enabling the removal of harmful pollutants and the recovery of valuable resources. In the latter, electrochemical catalysis is used to drive selective chemical transformations, opening up new pathways for the production of various chemicals and pharmaceuticals.The success of electrochemical catalysis is heavily dependent on the development of advanced catalytic materials and the optimization of the catalytic processes. Researchers in academia and industry are continuously exploring new strategies to design and synthesize highly active, selective, and durable catalysts, drawing inspiration from fields such as materials science, nanotechnology, and computational chemistry.One promising approach is the use of nanostructured materials, which offer a large surface area-to-volume ratio and the ability to fine-tune the electronic and structural properties of the catalysts. The incorporation of transition metals, noble metals, and their alloys into these nanostructured materials has led to significant improvements in catalytic performance, with researchers exploring innovative synthesis methods and novel catalyst architectures to further enhance activity and stability.Another area of active research is the development of non-precious metal-based catalysts, which aim to reduce the reliance on scarce and expensive precious metals, such as platinum and iridium. The exploration of earth-abundant elements, including iron, nickel, and cobalt, has yielded promising results, with researchers investigating ways to improve the catalytic activity and durability of these alternative materials.Computational modeling and simulation have also played a crucial role in the advancement of electrochemical catalysis. By coupling advanced computational techniques with experimental data, researchers can gain deeper insights into the underlying mechanisms of electrochemical reactions, enabling the rational design of more efficient and selective catalysts.As the world continues to grapple with the pressing challenges of energy security, environmental sustainability, and resource scarcity, the importance of electrochemical catalysis cannot be overstated. This transformative technology holds the potential to revolutionize the way we produce, store, and utilize energy, while also contributing to the development of more sustainable chemical processes and environmental remediation strategies.Through continued research, innovation, and collaboration amongscientists, engineers, and policymakers, the field of electrochemical catalysis is poised to play a pivotal role in shaping a more sustainable and prosperous future for our planet.。
电催化氧化处理苯酚废水

科学研究 2019,Vol.36No.11化学与生物工程 Chemistry&Bioengineering doi:10.3969/j.issn.1672-5425.2019.11.003电催化氧化处理苯酚废水[J].化学与生物工程,2019,36(11):1-4.ZHANG C,JIA Z Q,ZHAO Y X.Electrocatalytic oxidation treatment of phenol wastewater[J].Chemistry&Bioengineering,2019,36(11):1 -4.电催化氧化处理苯酚废水张 闯1,2,贾志奇1,3∗,赵永祥1,2∗(1.山西大学化学化工学院,山西太原030006;2.精细化学品教育部工程研究中心,山西太原030006;3.山西大学固废利用襄垣研发基地,山西太原030006)摘 要:采用固定尺寸的铱钌镀钛电极作阳极、不锈钢电极作阴极、锰炭复合材料作粒子电极,利用三维电极对苯酚模拟废水进行电催化降解,并考察了电压、不同电解质、氯化钠加量、反应时间等因素对苯酚模拟废水处理效果的影响。
得到最佳反应条件为:电压15V、氯化钠加量2g、反应时间120min。
在最佳条件下,填充10g粒子电极的三维电极处理100mL10000mg·L-1的苯酚模拟废水,苯酚转化率为96.10%,COD降解率达83.97%。
关键词:苯酚废水;电催化氧化;处理中图分类号:X703.1 文献标识码:A 文章编号:1672⁃5425(2019)11⁃0012⁃05Electrocatalytic Oxidation Treatment of Phenol WastewaterZHANG Chuang1,2,JIA Zhiqi1,3∗,ZHAO Yongxiang1,2∗(1.School of Chemistry and Chemical Engineering,Shanxi University,Taiyuan030006,China;2.Engineering Research Center for Fine Chemicals of Ministry of Education,Taiyuan030006,China;3.Xiangyuan Research and Development Base for Solid Waste Utilization,Shanxi University,Taiyuan030006,China)Abstract:Using a fixed-size titanium-plated titanium ruthenium electrode as an anode,a stainless steel electrode as a cathode,and a manganese-carbon composite material as a particle electrode,we performed the electrocatalytic degra⁃dation of phenol simulated wastewater by using the three-dimensional electrode,and investigated the effects of voltage, different electrolytes,sodium chloride dosage,and reaction time on the treatment efficiency of phenol simulated wastewater.The optimum reaction conditions are determined as follows:the voltage of15V,the sodium chloride dosage of 2g,and the reaction time of120min.By comparison with two-dimensional electrode and three-dimensional electrode, it is indicated that three-dimensional electrode is more efficient than two-dimensional electrode in the treatment of phenol simulated wastewater.Under optimum conditions,when100mL10000mgoL-1phenol simulated wastewater is treated by the three-dimensional electrode filled with10g particle electrode,the phenol conversion rate will reach96. 10%,and the COD degradation rate will reach83.97%.Keywords:phenol wastewater;electrocatalytic oxidation;treatment 在我国水污染控制中,含酚废水被列为重点解决的有害废水之一。
超级电容器文献综述
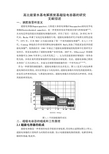
高比能量水基电解质炭基超级电容器的研究文献综述一、课题背景和意义超级电容器(Supercapacitor),又称超大容量电容器(Ultracapacitor)或电化学电容器(Electrochemical capacitor),是一种重要的电化学能量存储与转换装置[1-3]。
从双电层理论的提出到超级电容器的问世,经历了很长一段历史。
20世纪50年代末,Becke申请了双电层电容器的专利,超级电容器的研究才取得实质性进展[1]。
1971年,日本NEC公司成功制备了第一个商用超级电容器[4]。
在七八十年代,Conway和他的合作者利用赝电容电极材料RuO2制备了性能更加优异的超级电容器[5]。
美国政府在1989 年制定了超级电容器领域的短期和中长期研究计划项目,更是加速推动了超级电容器产业的发展。
2007年,《Discovery》将超级电容器列为2006年世界七大技术发现之一,认为其是能量储存领域的一项革命性发展,并将在某些重要领域替代传统能量存储装置。
至此,超级电容器已得到各界的广泛关注和认可,并成为全球新型储能器件的一个研究热点[3, 6-11]。
作为一种新型的储能器件,超级电容器具有众多优点,图1.1是其与电池和普通电容的对比情况。
对比结果显示与电池相比,超级电容器具有较低的能量密度,但是其功率密度较高;与普通电容相比,超级电容器具有较低的功率密度,但是其能量密度较高。
图1.1不同储能器件对比二、超级电容器的组成和工作原理2.1超级电容器的组成超级电容器是一种重要的电化学能量存储装置,其结构示意图如图2.1所示。
超级电容器的主要构件为对称的双电极、防止电极接触短路的隔膜、电解质和电极材料基底-集流体。
图2.1超级电容器的构成图示2.2工作原理基于超级电容器的不同构成材料及工作原理,可将其分为三类:(l)电化学双电层电容器(Electrochemical Double Layer capacitor,EDLC);(2)法拉第赝电容器(Faradic Pseudocapacitor,FPC);(3) 混合杂化型电容器(Hybrid Supercapacitor,HSC)。
2018年诺贝尔生理学或医学奖

2018年诺贝尔生理学或医学奖
2018年 10月 1日,诺贝尔奖评审委员会在瑞典卡罗林斯卡医学院宣布,将 2018年诺贝尔生理学或医学奖授予美国科学 家詹姆斯·艾里森(JamesP.Allison)和日本科学家本庶佑(TasukuHonjo),以表彰他们发现抑制负向免疫调控用于癌症治疗 的全新理论和方法。
本庶佑,1942年生于日本京都,现就职于日本京都大学。1992年,他首次发现另D1(Ishida等.TheEMBOJournal,1992)。随后大量的基础和临床实验证实,针对 PD1及其配体 PDL1通路的单克隆 抗体对多种肿瘤具有良好的治疗效果,目前临床广泛应用于实体瘤患者治疗的派姆单抗就是针对该通路。
frontalactivityrelatestointrospectionandactionbutnotto perception.JNeurosci,2014,341738~1747. 43 韩中胜,祁金顺.意识活动的神经生物学机制.生理科 学进展,2016,47339~345. 44 DehaeneS.Consciousnessandthebrain:Decipheringhow thebraincodesourthoughts.USA:PenguinBooks,2014, 161~199. 45 DehaeneS,ChangeuxJP.Experimentalandtheoreticalap proachestoconsciousprocessing. Neuron,2011,70 200~227. 46 LammeVA.Whyvisualattentionandawarenessarediffer ent.TrendsCognSci,2003,712~18. 47 LammeVA.How neurosciencewillchangeourview on consciousness.CognNeurosci,2010,1 204~220.
毕赤酵母表达手册

Pichia Expression KitVersion M01110225-0043Pichia Expression KitA Manual of Methods for Expression of Recombinant Proteins in Pichia pastorisCatalog no. K1710-01tech_service@iiINDIVIDUAL PICHIA EXPRESSION KIT LICENSE AGREEMENTThe Pichia Expression Kit is based on the yeast Pichia pastoris. Pichia pastoris was developed into an expression system by scientists at Salk Institute Biotechnology/Industry Associates (SIBIA) for high-level expression of recombinant proteins. All patents for Pichia pastoris and licenses for its use as an expression system are owned by Research Corporation Technologies, Inc. Tucson, Arizona. Invitrogen has an exclusive license to sell the Pichia Expression Kit to scientists for research purposes only, under the terms described below. Use of Pichia pastoris by commercial corporations requires the user to obtain a commercial license as detailed below. Before using the Pichia Expression Kit, please read the following license a greement. If you do not agree to be bound by its terms, contact Invitrogen within 10 days for authorization to return the unused Pichia Expression Kit and to receive a full credit. If you do agree to the terms of this Agreement, please complete the User Registration Card and return it to Invitrogen before using the kit.INDIVIDUAL PICHIA EXPRESSION KIT LICENSE AGREEMENTInvitrogen Corporation (INVITROGEN) grants you a non-exclusive license to use the enclosed Pichia Expression Kit (EXPRESSION KIT) for academic research or for evaluation purposes only. The EXPRESSION KIT is being transferred to you in furtherance of, and reliance on, such license. You may not use the EXPRESSION KIT, or the materials contained therein, for any commercial purpose without a license for such purpose from RESEARCH CORPORATION TECHNOLOGIES, INC., Tucson, Arizona. Commercial purposes include the use in or sale of expressed proteins as a commercial product, or use to facilitate or advance research or development of a commercial product. Commercial entities may conduct their evaluation for one year at which time this license automatically terminates. Commercial entities will be contacted by Research Corporation Technologies during the evaluation period regarding the purchase of a commercial license.Access to the EXPRESSION KIT must be limited solely to those officers, employees and students of your institution who need access thereto in order to perform the above-described research or evaluation. You must inform each of such officer, employee and student of the provisions of this Agreement and require them to agree, in writing, to be bound by the provisions of this Agreement. You may not distribute the EXPRESSION KIT to others, even those within your own institution. You may transfer modified, altered or original material from the EXPRESSION KIT to a third party following notification of INVITROGEN such that the recipient can be licensed. You may not assign, sub-license, rent lease or otherwise transfer this License or any of the rights or obligation hereunder, except as expressly permitted.This License is effective until terminated. You may terminate it at any time by destroying all Pichia expression products in your control. It will also terminate automatically if you fail to comply with the terms and conditions of the Agreement. You shall, upon termination of the License, destroy all Pichia Expression Kits in your control, and so notify INVITROGEN in writing.This License Shall be governed in its interpretation and enforcement by the laws of the State of California.Product User Registration CardPlease complete and return the enclosed Product User Registration Card for each Pichia Expression Kit that you purchase. This will serve as a record of your purchase and registration and will allow Invitrogen to provide you with technical support and manual updates. It will also allow Invitrogen to update you on future developments of and improvements to the Pichia Expression Kit. The agreement outlined above becomes effective upon our receipt of your User Registration Card or 10 days following the sale of the Pichia Expression Kit to you. Use of the kit at any time results in immediate obligation to the terms and conditions stated in this Agreement.Technical ServicesInvitrogen provides Technical Services to all of our registered Pichia Expression Kit users. Please contact us if you need assistance with the Pichia Expression Kit.United States Headquarters:Japanese Headquarters European Headquarters:Invitrogen Corporation1600 Faraday AvenueCarlsbad, CA 92008 USATel: 1 760 603 7200Tel (Toll Free): 1 800 955 6288 Fax: 1 760 602 6500E-mail:tech_service@ Invitrogen Japan K.K.Nihonbashi Hama-Cho Park Bldg. 4F2-35-4, Hama-Cho, NihonbashiTel: 81 3 3663 7972Fax: 81 3 3663 8242E-mail: jpinfo@Invitrogen Ltd3 Fountain DriveInchinnan Business ParkPaisley PA4 9RF, UKTel (Free Phone Orders): 0800 269 210Tel (General Enquiries): 0800 5345 5345Fax: +44 (0) 141 814 6287E-mail: eurotech@iiiivTable of ContentsMaterials (vii)Purchaser Notification (x)Product Qualification (xii)Introduction (1)Overview (1)Experimental Outline (3)Recombination and Integration in Pichia (7)Methods (11)Pichia Strains (11)E. coli Strains (13)Selecting a Pichia Expression Vector (14)pHIL-D2 (16)pPIC3.5 (17)pHIL-S1 (18)pPIC9 (19)Signal Sequence Processing (20)Cloning into the Pichia Expression Vectors (21)Transformation into E. coli (26)Preparation of Transforming DNA (27)Growth of Pichia for Spheroplasting (30)Preparation of Spheroplasts (32)Transformation of Pichia (34)Screening for Mut+ and Mut S Transformants (36)PCR Analysis of Pichia Integrants (40)Expression of Recombinant Pichia Strains (42)Analysis by SDS-Polyacrylamide Gel Electrophoresis (45)Optimization of Pichia Protein Expression (47)Scale-up of Expression (49)Protein Purification and Glycosylation (51)Recipes (53)E. coli Media Recipes (53)Pichia Media Recipes (54)Appendix (59)Electroporation of Pichia (59)PEG 1000 Transformation Method for Pichia (60)Lithium Chloride Transformation Method (61)Total DNA Isolation from Pichia (62)Detection of Multiple Integration Events (63)Procedure for Total RNA Isolation from Pichia (64)β-Galactosidase Assay (65)Technical Service (67)References (69)vviMaterialsKit Contents Box 1: Spheroplast Module. Store at room temperature.Reagent Amount ComponentsSOS media 20 ml 1 M Sorbitol0.3X YPD10 mM CaCl2Sterile Water 2 x 125 ml Autoclaved, deionized waterSE 2 x 125 ml 1 M Sorbitol25 mM EDTA, pH 8.0SCE 2 x 125 ml 1 M Sorbitol10 mM Sodium citrate buffer, pH 5.81 mM EDTA1 M Sorbitol2 x 125 ml --CaS 2 x 60 ml 1 M Sorbitol10 mM Tris-HCl, pH 7.5;10 mM CaCl240% PEG 25 ml 40% (w/v) PEG 3350 (Reagent grade) in waterCaT 25 ml 20 mM Tris-HCl, pH 7.520 mM CaCl2Stab Vials: Pichia and E. coli stabs. Store at +4°C.Phenotype(Pichia only)GenotypeStrain Amountstab his4Mut+GS115 1stab arg4 his4 aox1::ARG4 Mut S, Arg+KM71 1GS115 Albumin 1 stab HIS4Mut SGS115 β-Gal 1 stab HIS4Mut+stab F´ {pro AB, lac I q, lac Z∆M15, Tn10 (Tet R)} mcr A,TOP10F´ 1∆(mrr-hsd RMS-mcr BC), φ80lac Z∆M15, ∆lac X74,deo R, rec A1, ara D139, ∆(ara-leu)7697, gal U,gal K, rps L (Str R), end A1, nup G λ-.Box 2: Spheroplast Module. Store at -20°C.ComponentsReagent AmountZymolyase 10 x 20 µl 3 mg/ml Zymolyase in water(100,000 units/g lytic activity)1 M DTT 10 x 1 ml 1 M dithiothreitol in watercontinued on next pageviiKit Contents,continuedVector Box. Store at -20°C.Reagent DescriptionpHIL-D210 µg, lyophilized in TE, pH 8.0Vector for intracellular expression in PichiapPIC3.510 µg, lyophilized in TE, pH 8.0Vector for intracellular expression in PichiapHIL-S110 µg, lyophilized in TE, pH 8.0 Vector for secreted expression in Pichia. Uses the PHO1 signal sequencepPIC910 µg, lyophilized in TE, pH 8.0 Vector for secreted expression in Pichia. Uses the α-factor signal sequencePrimer Box. Store at -20°C.5´ AOX1 sequencing primer2 µg (312 pmoles), lyophilized5´-GACTGGTTCCAATTGACAAGC-3´3´ AOX1 sequencing primer2 µg (314 pmoles), lyophilized5´-GCAAATGGCATTCTGACATCC-3´α-Factor sequencing primer2 µg (315 pmoles), lyophilized5´-TACTATTGCCAGCATTGCTGC-3´Media The following prepackaged media is included for your convenience. Instructions for use are provided on the package.Media Amount Yield YP Base Medium 2 pouches 2 liters of YP mediumYP Base Agar Medium 2 pouches 2 liters of YP mediumYeast Nitrogen Base 1 pouch 500 ml of 10X YNBFor transformation of Pichia by spheroplasting, the Pichia Spheroplast Module isavailable separately from Invitrogen (see below for ordering information).Product Reactions or Amount Catalog no.Pichia Spheroplast Module 10 spheroplast preparations(50 transformations)K1720-01continued on next pageviiiRequired Equip-ment and Supplies (not provided) • 30°C rotary shaking incubator• Water baths capable of 37°C, 45°C, and 100°C• Centrifuge suitable for 50 ml conical tubes (floor or table-top)• Baffled culture flasks with metal covers (50 ml, 250 ml, 500 ml, 1000 ml, and 3 L)• 50 ml sterile, conical tubes• 6 ml and 15 ml sterile snap-top tubes (Falcon 2059 or similar)• UVSpectrophotometer• Mini agarose gel apparatus and buffers• Polyacrylamide Gel Electrophoresis apparatus and buffers• Media for transformation, growth, screening, and expression (see Recipes, pages 53-58) • 5% SDS solution (10 ml per transformation)• Sterile cheesecloth or gauze• Breaking Buffer (see Recipes, page 58)• Acid-washed glass beads (available from Sigma)• Replica-plating equipment (optional)• BeadBreaker™ (optional)ixPurchaser NotificationIntroduction The Pichia Expression Kit is based on the yeast Pichia pastoris. Pichia pastoris wasdeveloped into an expression system by scientists at Salk Institute Biotechnology/ IndustryAssociates (SIBIA) and Phillips Petroleum for high-level expression of recombinantproteins. All patents for Pichia pastoris and licenses for its use as an expression system areowned by Research Corporation Technologies (RCT), Inc., Tucson, Arizona. Forinformation on commercial licenses, please see page x.The Nature of the Invitrogen License Invitrogen has an exclusive license to sell the Pichia Expression Kit to scientists for research purposes only, under the terms described below. Use of Pichia pastoris by commercial entities for any commercial purpose requires the user to obtain a commercial license as detailed below. Before using the Pichia Expression Kit, please read the following license agreement. If you do not agree to be bound by its terms, contact Invitrogen within 10 days for authorization to return the unused Pichia Expression Kit and to receive a full credit. If you do agree to the terms of this license agreement, please complete the User Registration Card and return it to Invitrogen before using the kit.Pichia pastoris Patents Pichia pastoris is covered by one or more of the following U.S. patents and corresponding foreign patents owned and licensed by Research Corporation Technologies:4,683,293 4,808,537 4,812,405 4,818,700 4,837,148 4,855,231 4,857,467 4,879,231 4,882,279 4,885,242 4,895,800 4,929,555 5,002,876 5,004,688 5,032,516 5,122,465 5,135,868 5,166,329Individual Pichia Expression Kit License Agreement Invitrogen Corporation ("Invitrogen") grants you a non-exclusive license to use the enclosed Pichia Expression Kit ("Expression Kit") for academic research or for evaluation purposes only. The Expression Kit is being transferred to you in furtherance of, and reliance on, such license. You may not use the Expression Kit, or the materials contained therein, for any commercial purpose without a license for such purpose from Research Corporation Technologies, Inc., Tucson, Arizona.Definition of Commercial Purpose Commercial purposes include:(a) any use of Expression Products in a Commercial Product(b) any use of Expression Products in the manufacture of a Commercial Product(c) any sale of Expression Products(d) any use of Expression Products or the Expression Kit to facilitate or advanceresearch or development of a Commercial Product(e) any use of Expression Products or the Expression Kit to facilitate or advance anyresearch or development program the results of which will be applied to thedevelopment of Commercial Products"Expression Products" means products expressed with the Expression Kit, or with the use of any vectors or host strains in the Expression Kit. "Commercial Product" means any product intended for sale or commercial use.Commercial entities may conduct their evaluation for one year at which time this license automatically terminates. Research Corporation Technologies will contact commercial entities during the evaluation period regarding their desire for a commercial license.continued on next pagexPurchaser Notification, continuedIndividual Responsibilities Access to the Expression Kit must be limited solely to those officers, employees and students of your institution who need access to perform the above-described research or evaluation. You must inform each such officer, employee and student of the provisions of this license agreement and require them to agree, in writing, to be bound by the provisions of this license agreement. You may not distribute neither the Expression Kit nor the vectors or host strains contained in it to others, even to those within your own institution. You may only transfer modified, altered, or original material from the Expression Kit to a third party following written notification of, and written approval from, Invitrogen so that the recipient can be licensed. You may not assign, sub-license, rent, lease or otherwise transfer this license agreement or any of the rights or obligation thereunder, except as expressly permitted by Invitrogen and RCT.Termination of License This license agreement is effective until terminated. You may terminate it at any time by destroying all Pichia expression products in your control. It will also terminate auto-matically if you fail to comply with the terms and conditions of the license agreement. You shall, upon termination of the license agreement, destroy all Pichia Expression Kits in your control, and so notify Invitrogen in writing.This License shall be governed in its interpretation and enforcement by the laws of the State of California.Contact for Commercial Licensing Bennett Cohen, Ph.D.Research Corporation Technologies 101 North Wilmot Road, Suite 600 Tucson, Arizona 85711-3335 Phone: (520) 748-4400Fax: (520)748-0025User Registration Card Please complete and return the enclosed User Registration Card for each PichiaExpression Kit that you purchase. This will serve as a record of your purchase and regis-tration and will allow Invitrogen to provide you with technical support and manualupdates. It will also allow Invitrogen to update you on future developments and improve-ments to the Pichia Expression Kit. The agreement outlined above becomes effectiveupon our receipt of your User Registration Card or 10 days following the sale of thePichia Expression Kit to you. Use of the kit at any time results in immediate obligation tothe terms and conditions stated in this license agreement.xiProduct QualificationIntroduction This section describes the criteria used to qualify the components in the PichiaExpression Kit.Vectors All expression vectors are qualified by restriction enzyme digestion. Restriction digests must demonstrate the correct banding pattern when electrophoresed on an agarose gel.Spheroplast Reagents The spheroplast reagents are qualified by spheroplast preparation of GS115 following the protocol provided in the Pichia Expression Kit manual. At least 70% of the Pichia pastoris cells must form spheroplasts in 30 minutes or less.Pichia Strains The Pichia strains are by demonstrating viability of the culture. Single colonies should arise within 48 hours after streaking on YPD medium from the stabPrimers Sequencing primers are lot tested by automated DNA sequencing experiments.Buffers andSolutionsAll buffers and solutions are extensively tested for sterility.Media All Pichia growth and expression media are qualified by growing the GS115 Pichiastrain.xiiIntroductionOverviewReview Articles The information presented here is designed to give you a concise overview of the Pichia pastoris expression system. It is by no means exhaustive. For further information, pleaseread the articles cited in the text along with recent review articles (Buckholz and Gleeson,1991; Cregg et al., 1993; Sreekrishna et al., 1988; Wegner, 1990). A general review offoreign gene expression in yeast is also available (Romanos et al., 1992).General Characteristics of Pichia pastoris As a eukaryote, Pichia pastoris has many of the advantages of higher eukaryotic expression systems such as protein processing, protein folding, and posttranslational modification, while being as easy to manipulate as E. coli or Saccharomyces cerevisiae. It is faster, easier, and less expensive to use than other eukaryotic expression systems such as baculovirus or mammalian tissue culture, and generally gives higher expression levels. As a yeast, it shares the advantages of molecular and genetic manipulations with Saccharomyces, and has the added advantage of 10- to 100-fold higher heterologous protein expression levels. These features make Pichia very useful as a protein expression system.Similarity to Saccharomyces Many of the techniques developed for Saccharomyces may be applied to Pichia including: • transformation by complementation• genedisruption• genereplacementIn addition, the genetic nomenclature used for Saccharomyces has been applied to Pichia. For example, the HIS4 gene in both Saccharomyces and Pichia encodes histidinol dehydrogenase. There is also cross-complementation between gene products in both Saccharomyces and Pichia. Several wild-type genes from Saccharomyces complement comparable mutant genes in Pichia. Genes such as HIS4, LEU2, ARG4, TRP1, and URA3 all complement their respective mutant genes in Pichia.Pichia pastoris as a Methylotrophic Yeast Pichia pastoris is a methylotrophic yeast, capable of metabolizing methanol as its sole carbon source. The first step in the metabolism of methanol is the oxidation of methanol to formaldehyde using molecular oxygen by the enzyme alcohol oxidase. This reaction generates both formaldehyde and hydrogen peroxide. To avoid hydrogen peroxide toxicity, methanol metabolism takes place within a specialized cell organelle called the peroxisome, which sequesters toxic by-products from the rest of the cell. Alcohol oxidase has a poor affinity for O2, and Pichia pastoris compensates by generating large amounts of the enzyme. The promoter regulating the production of alcohol oxidase drives heterologous protein expression in Pichia.Two Alcohol Oxidase Proteins The AOX1 and AOX2 genes code for alcohol oxidase in Pichia pastoris. The AOX1 gene product accounts for the majority of alcohol oxidase activity in the cell. Expression of the AOX1 gene is tightly regulated and induced by methanol to high levels, typically > 30% ofthe total soluble protein in cells grown with methanol as the carbon source. The AOX1 gene has been isolated and the AOX1 promoter is used to drive expression of the gene of interest (Ellis et al., 1985; Koutz et al., 1989; Tschopp et al., 1987a). While AOX2 is about 97% homologous to AOX1, growth on methanol is much slower than with AOX1. This slowgrowth allows isolation of Mut S strains (aox1) (Cregg et al., 1989; Koutz et al., 1989).continued on next page1Overview, continuedExpression Expression of the AOX1 gene is controlled at the level of transcription. In methanol-grown cells approximately 5% of the polyA+ RNA is from the AOX1 gene. The regulation of theAOX1 gene is a two step process: a repression/derepression mechanism plus an inductionmechanism (e.g. GAL1 gene in Saccharomyces (Johnston, 1987)). Briefly, growth onglucose represses transcription, even in the presence of the inducer methanol. For thisreason, growth on glycerol is recommended for optimal induction with methanol. Pleasenote that growth on glycerol (derepression) is not sufficient to generate even minute levelsof expression from the AOX1 gene. The inducer, methanol, is necessary for detectablelevels of AOX1 expression (Ellis et al., 1985; Koutz et al., 1989; Tschopp et al., 1987a).Phenotype of aox1 mutants Loss of the AOX1 gene, and thus a loss of most of the cell's alcohol oxidase activity, results in a strain that is phenotypically Mut S (Methanol utilization slow). This has in the past been referred to as Mut. The Mut S designation has been chosen to accurately describe the phenotype of these mutants. This results in a reduction in the cells' ability to metabolize methanol. The cells, therefore, exhibit poor growth on methanol medium. Mut+ (Methanol utilization plus) refers to the wild type ability of strains to metabolize methanol as the sole carbon source. These two phenotypes are used when evaluating Pichia transformants for integration of your gene (Experimental Outline, page 3).Intracellular and Secretory Protein Expression Heterologous expression in Pichia can be either intracellular or secreted. Secretion requires the presence of a signal sequence on the expressed protein to target it to the secretory pathway. While several different secretion signal sequences have been used successfully, including the native secretion signal present on some heterologous proteins, success has been variable. The secretion signal sequence from the Saccharomyces cerevisiaeα factor prepro peptide has been used most successfully (Cregg et al., 1993; Scorer et al., 1993).The major advantage of expressing heterologous proteins as secreted proteins is that Pichia pastoris secretes very low levels of native proteins. That, combined with the very low amount of protein in the minimal Pichia growth medium, means that the secreted heterologous protein comprises the vast majority of the total protein in the medium and serves as the first step in purification of the protein (Barr et al., 1992). Note: If there are recognized glycosylation sites (Asn-X-Ser/Thr) in your protein's primary sequence, glycosylation may occur at these sites.Posttranslational Modifications In comparison to Saccharomyces cerevisiae, Pichia may have an advantage in the glyco-sylation of secreted proteins because it may not hyperglycosylate. Both Saccharomyces cerevisiae and Pichia pastoris have a majority of N-linked glycosylation of the high-mannose type; however, the length of the oligosaccharide chains added posttranslationally to proteins in Pichia (average 8-14 mannose residues per side chain) is much shorter than those in S. cerevisiae (50-150 mannose residues) (Grinna and Tschopp, 1989; Tschopp et al., 1987b). Very little O-linked glycosylation has been observed in Pichia.In addition, Saccharomyces cerevisiae core oligosaccharides have terminal α1,3 glycan linkages whereas Pichia pastoris does not. It is believed that the α1,3 glycan linkages in glycosylated proteins produced from Saccharomyces cerevisiae are primarily responsible for the hyper-antigenic nature of these proteins making them particularly unsuitable for therapeutic use. Although not proven, this is predicted to be less of a problem for glycoproteins generated in Pichia pastoris, because it may resemble the glycoprotein structure of higher eukaryotes (Cregg et al., 1993).2Experimental OutlineSelection of Vector and Cloning To utilize the strong, highly inducible P AOX1 promoter for expression of your protein, four expression vectors are included in this kit. pHIL-D2 and pPIC3.5 are used for intracellular expression while pHIL-S1 and pPIC9 are used for secreted expression (see pages 14-19 for more information). Before cloning your insert, you must...• decide whether you want intracellular or secreted expression.• analyze your insert for the following restriction sites: Sac I, Stu I, Sal I, Not I, and Bgl II. These sites are recommended for linearizing your construct prior to Pichiatransformation. If your insert has all of these sites, see pages 28-29 for alternate sites.Transformation and IntegrationTwo different phenotypic classes of His+ recombinant strains can be generated: Mut+ and Mut S. Mut S refers to the "Methanol utilization slow" phenotype caused by the loss of alcohol oxidase activity encoded by the AOX1 gene. A strain with a Mut S phenotype has a mutant aox1 locus, but is wild type for AOX2. This results in a slow growth phenotype on methanol medium. Transformation of strain GS115 can yield both classes of transformants, His+ Mut+ and His+Mut S, while KM71 yields only His+ Mut S since the strain itself is Mut S. Both Mut+ and Mut S recombinants are useful to have as one phenotype may favor better expression of your protein than the other. Due to clonal variation, you should test 6-10 recombinants per phenotype. There is no way to predict beforehand which construct or isolate will better express your protein. We strongly recommend that you analyze Pichia recombinants by PCR to confirm integration of your construct (see page 40).Once you have successfully cloned your gene, you will then linearize your plasmid to stimulate recombination when the plasmid is transformed into Pichia. The table below describes the types of recombinants you will get by selective digestion of your plasmid. RestrictionEnzymeIntegration Event GS115 Phenotype KM71 PhenotypeSal I or Stu I Insertion at his4His+ Mut+ His+ Mut SSac I Insertion at 5´AOX1 regionHis+ Mut+ His+ Mut SNot I or Bgl II Replacement atAOX1 locusHis+ Mut SHis+ Mut+His+ Mut S (notrecommended, see page 11)Expression and Scale-up After confirming your Pichia recombinants by PCR, you will test expression of both His+Mut+ and His+ Mut S recombinants. This will involve growing a small culture of each recombinant, inducing with methanol, and taking time points. If looking for intracellular expression, analyze the cell pellet from each time point by SDS polyacrylamide gel electrophoresis (SDS-PAGE). If looking for secreted expression, analyze both the cellpellet and supernatant from each time point. We recommend that you analyze your SDS-PAGE gels by both Coomassie staining and Western blot, if you have an antibody to your protein. We also suggest checking for protein activity by assay, if one is available. Not all proteins express to the level of grams per liter, so it is advisable to check by Western blotor activity assay, and not just by Coomassie staining of SDS-PAGE gels for production of your protein.Choose the Pichia recombinant strain that best expresses your protein and optimizeinduction based on the suggestions on pages 47-48. Once expression is optimized, scale-up your expression protocol to produce more protein.continued on next page3。
电化学吸附英文
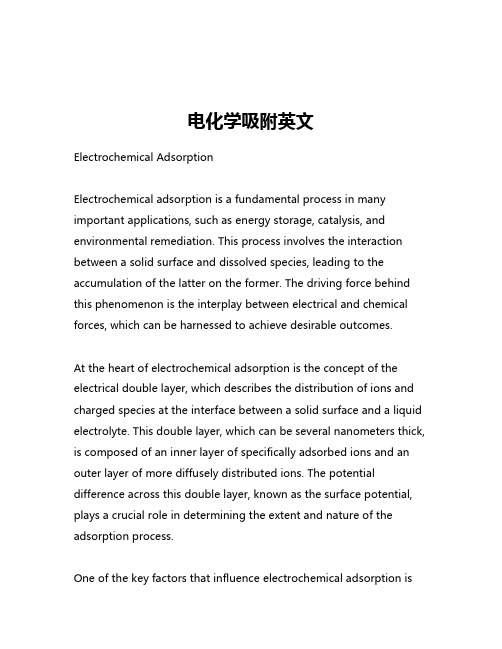
电化学吸附英文Electrochemical AdsorptionElectrochemical adsorption is a fundamental process in many important applications, such as energy storage, catalysis, and environmental remediation. This process involves the interaction between a solid surface and dissolved species, leading to the accumulation of the latter on the former. The driving force behind this phenomenon is the interplay between electrical and chemical forces, which can be harnessed to achieve desirable outcomes.At the heart of electrochemical adsorption is the concept of the electrical double layer, which describes the distribution of ions and charged species at the interface between a solid surface and a liquid electrolyte. This double layer, which can be several nanometers thick, is composed of an inner layer of specifically adsorbed ions and an outer layer of more diffusely distributed ions. The potential difference across this double layer, known as the surface potential, plays a crucial role in determining the extent and nature of the adsorption process.One of the key factors that influence electrochemical adsorption isthe surface charge of the solid material. Depending on the pH of the solution and the point of zero charge (PZC) of the solid, the surface can be positively or negatively charged. This surface charge, in turn, affects the adsorption of ions and molecules from the solution. For example, if the surface is positively charged, it will preferentially adsorb anions from the solution, while a negatively charged surface will attract cations.The strength of the adsorption interaction is also influenced by the chemical nature of the adsorbate and the adsorbent. Specific interactions, such as hydrogen bonding, ion-dipole interactions, and van der Waals forces, can all contribute to the overall adsorption energy. Additionally, the morphology and surface area of the adsorbent material can play a significant role in the adsorption capacity and kinetics.One of the key applications of electrochemical adsorption is in the field of energy storage. In electrochemical capacitors, also known as supercapacitors, the storage of energy is achieved through the reversible adsorption and desorption of ions at the electrode-electrolyte interface. The high surface area of the electrode materials, combined with the rapid kinetics of the adsorption process, allows for the development of high-power energy storage devices with long cycle life.Another important application of electrochemical adsorption is in the area of catalysis. Many catalytic processes, such as fuel cell reactions and electrochemical water splitting, involve the adsorption of reactants and intermediates on the catalyst surface. The controlled adsorption of these species can enhance the catalytic activity and selectivity, leading to improved efficiency and performance.Environmental remediation is yet another field where electrochemical adsorption plays a crucial role. The removal of heavy metals, organic pollutants, and other contaminants from water and wastewater can be achieved through the adsorption of these species onto electrode materials. The ability to tune the surface properties of the adsorbent, as well as the application of an external electric field, can enhance the selectivity and efficiency of the adsorption process.In addition to these well-established applications, electrochemical adsorption is also being explored in emerging fields, such as electrochemical sensors, energy harvesting, and biomedical applications. The versatility and tunability of this process make it a valuable tool in the development of innovative technologies.To further advance the understanding and application of electrochemical adsorption, ongoing research is focused on several key areas. These include the development of novel adsorbent materials with tailored surface properties, the investigation of thefundamental mechanisms governing the adsorption process, and the optimization of the operating conditions and system design for various applications.In conclusion, electrochemical adsorption is a complex and multifaceted phenomenon that underpins a wide range of important technologies. By harnessing the interplay between electrical and chemical forces, researchers and engineers can harness the power of this process to address pressing challenges in energy, environment, and beyond. As our understanding of electrochemical adsorption continues to deepen, we can expect to see even more innovative applications emerge in the years to come.。
电复律的英语
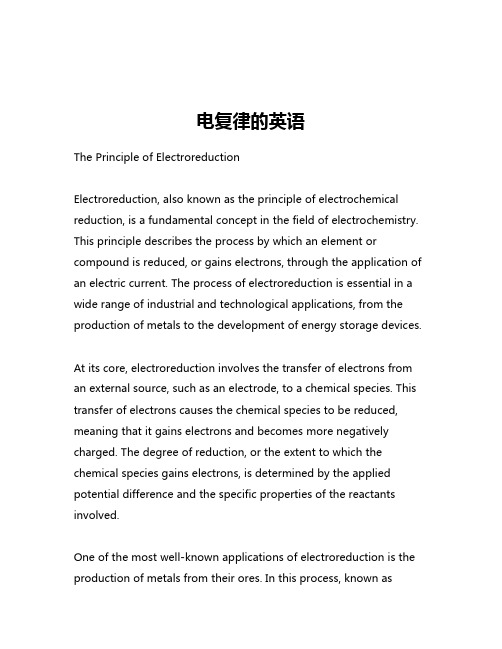
电复律的英语The Principle of ElectroreductionElectroreduction, also known as the principle of electrochemical reduction, is a fundamental concept in the field of electrochemistry. This principle describes the process by which an element or compound is reduced, or gains electrons, through the application of an electric current. The process of electroreduction is essential in a wide range of industrial and technological applications, from the production of metals to the development of energy storage devices.At its core, electroreduction involves the transfer of electrons from an external source, such as an electrode, to a chemical species. This transfer of electrons causes the chemical species to be reduced, meaning that it gains electrons and becomes more negatively charged. The degree of reduction, or the extent to which the chemical species gains electrons, is determined by the applied potential difference and the specific properties of the reactants involved.One of the most well-known applications of electroreduction is the production of metals from their ores. In this process, known aselectrochemical metal extraction, an electric current is used to reduce metal ions in a solution or molten salt to their metallic form. This process is particularly important for the production of metals such as aluminum, copper, and zinc, which are essential for a wide range of industrial and technological applications.Another important application of electroreduction is in the field of energy storage. Electrochemical energy storage devices, such as batteries and fuel cells, rely on the principle of electroreduction to store and release energy. In these devices, the reduction of chemical species, such as oxygen or hydrogen, is used to generate an electric current that can be used to power various electronic devices or systems.The principle of electroreduction is also crucial in the field of environmental remediation. In this context, electroreduction can be used to remove or transform harmful pollutants, such as heavy metals or organic compounds, from contaminated water or soil. By applying an electric current, the pollutants can be reduced and converted into less harmful or more easily removable forms.In addition to these practical applications, the principle of electroreduction is also of great importance in the field of analytical chemistry. Electrochemical techniques, such as voltammetry and potentiometry, rely on the principles of electroreduction to analyzethe composition and properties of various chemical species.Overall, the principle of electroreduction is a fundamental concept in electrochemistry that has a wide range of practical applications across various industries and fields of study. From the production of metals to the development of energy storage devices and environmental remediation, the ability to control and harness the process of electroreduction has been instrumental in driving technological progress and addressing important societal challenges.。
双目立体视觉 综述

•
•
[13] 常淑华.双目立体视觉匹配的预处理技术[J].吉林大学学报(理学版),2012,50(1):95-100
[14] 杨明.基于双目立体视觉的三维重建研究[D].武汉工程大学,2012 2013-11-5 2
参考文献(二)
• • • [15] 尚倩,阮秋琦等.双目立体视觉的目标识别与定位[J].智能系统学报,2011,6(4):303-311 [16] 罗丹,廖志贤.基于OpenCV的双目立体视觉测距[J].大众科技,2011(4):52-71 [17] 万智萍 基于OpenCV的双目立体视觉监控跟踪系统_
•
• •
[18] 何春燕,周付根.双目立体视觉系统定标误差分析[J].中国体视与图像分析,2003,8(4):230-234
[19] 倪厚强,卞德广等.双目立体视觉运动机构的设计[J].机械设计与研究,2008,,24(6):117-120 [20] 刘金颂,原思聪等.双目立体视觉中的摄像机标定技术研究[J].计算机工程与应用,2008,44(6):237239
一、研究意义
1、什么是视觉?
2、什么是计算机视觉?
3、什么是双目立体视觉?
2013-11-5
5
“双目”对于“单目”的优势
当一个摄像机拍摄图像时,由于图像中的像素点坐标 相对于真实的世界坐标并不是唯一的,这就造成深度信息 的丢失。
然而用两个摄像机同时拍摄图像时,可以获取同一场
景的两幅不同的图像,通过三角测量原理计算图像像素间
计算机视觉
双目立体视觉 Binocular stereo vision
周杉 2013.10.24
2013-11-5 1
参考文献(一)
• • • • [1] 隋婧金,金伟其.双目立体视觉技术的实现及其进展 [J].电子技术应用 ,2004(10) [2] 丁香,韩蕊,张岁.浅析双目立体视觉技术及其发展[J] .华人时刊(中旬刊),2012(12) [3] 牛海涛 .双目立体视觉关键技术研究[D]. 苏州大学:光学 ,2011 [4] 程黄金.双目立体视觉系统的技术分析与应用前景[J].Computer Knowledge and Technology,2011:21452147 • • • • [5] 郑俊.基于双目立体视觉的机器人测量技术研究[J] . 计量技术,2005:15-17 [6] 刘天亮.双目立体视觉的若干理论问题研究及应用[D].东南大学,2009 [7] 葛动元,姚锡凡等.双目立体视觉系统的标定[J].机械设计与制造,2010(6):188-189 [8] 张可,张棉好.双目立体视觉系统的非线性模型建立[J].传感器与微笑系统,2006,25(10):31-37 ISTIC、PKU
心理学英语介绍
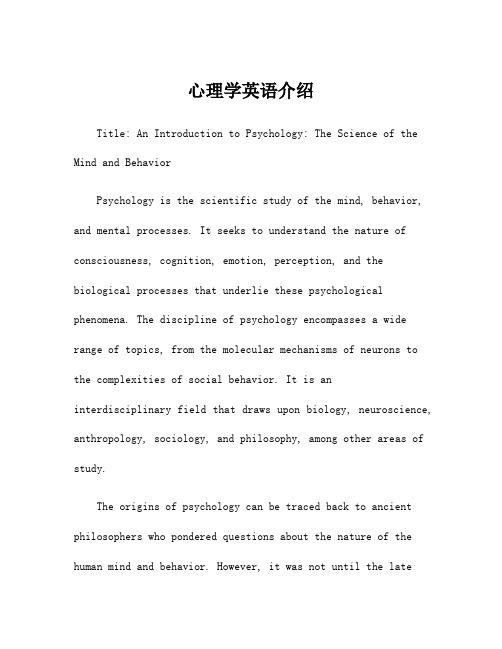
心理学英语介绍Title: An Introduction to Psychology: The Science of the Mind and BehaviorPsychology is the scientific study of the mind, behavior, and mental processes. It seeks to understand the nature of consciousness, cognition, emotion, perception, and the biological processes that underlie these psychological phenomena. The discipline of psychology encompasses a wide range of topics, from the molecular mechanisms of neurons to the complexities of social behavior. It is aninterdisciplinary field that draws upon biology, neuroscience, anthropology, sociology, and philosophy, among other areas of study.The origins of psychology can be traced back to ancient philosophers who pondered questions about the nature of the human mind and behavior. However, it was not until the late19th century that psychology began to emerge as a distinct scientific discipline. Wilhelm Wundt, considered the fatherof modern psychology, established the first psychological laboratory in Leipzig, Germany in 1879. Since then,psychology has evolved into a diverse field with various branches, including cognitive psychology, clinical psychology, social psychology, developmental psychology, and many others.Cognitive psychology focuses on the study of mental processes, such as perception, memory, language, thought, and problem-solving. Researchers in this area aim to understand how people acquire, process, store, and retrieve information from their environment. One of the key findings in cognitive psychology is the concept of working memory, which refers to the limited capacity system responsible for temporary storage and manipulation of information necessary for comprehension, learning, and reasoning.Clinical psychology, on the other hand, is concerned with the diagnosis, treatment, and prevention of mental disorders and emotional problems. Clinicians work with individuals, families, and groups to help them cope with and overcome psychological difficulties. They use various therapeutic approaches, such as psychoanalysis, behavioral therapy, cognitive-behavioral therapy, and humanistic therapy, depending on the needs of their clients.Social psychology explores the ways in which people's thoughts, feelings, and behaviors are influenced by the presence and actions of others. This branch of psychology investigates topics such as attitudes, beliefs, group dynamics, conformity, prejudice, and interpersonal attraction. Social psychologists also study the factors that contributeto prosocial behavior, such as altruism, cooperation, and helping.Developmental psychology examines changes in behavior and psychological processes over the lifespan. Researchers inthis field investigate how genetic and environmental factors interact to shape the course of human development. They study the physical, cognitive, social, and emotional growth of individuals from infancy through old age. One of the key concepts in developmental psychology is the idea of critical periods, which refers to specific times during development when certain skills or abilities are most easily acquired.Neuropsychology is another important branch of psychology that focuses on the relationship between brain function and behavior. Neuropsychologists study the effects of brain damage or disease on cognitive and emotional processes. They use techniques such as brain imaging, electrophysiological recording, and behavioral testing to assess the neural correlates of various psychological functions.In recent years, the field of psychology has been transformed by advances in technology and research methods. For example, functional magnetic resonance imaging (fMRI) has allowed researchers to observe brain activity in real-time, providing insights into the neural mechanisms underlying complex behaviors and emotions. Similarly, the availability of large datasets and sophisticated statistical tools has enabled psychologists to conduct more rigorous and precise studies than ever before.Despite its many achievements, psychology still faces numerous challenges and controversies. One major issue is the tension between the desire for scientific rigor and the need to address complex, real-world problems. Some critics argue that psychology has become too focused on laboratory experiments and statistical analyses at the expense of understanding human experience in its full richness and complexity. Others contend that psychology has been too quick to embrace reductionist explanations that emphasizebiological or genetic factors while neglecting the role of culture, history, and individual agency.Another challenge facing psychology is the issue of replicability and validity in research. In recent years, several high-profile studies have failed to replicate previous findings, casting doubt on the reliability of some psychological research. This has led to increased scrutiny of research methods and a call for greater transparency and openness in data sharing and analysis.Furthermore, psychology faces ethical challenges related to the protection of research participants, the use of deception in experiments, and the potential misuse of psychological knowledge for harmful purposes. As psychologists gain access to increasingly sensitive personal information through technologies such as online surveys and social media, they must grapple with issues of privacy, confidentiality, and informed consent.Despite these challenges, psychology remains a dynamic and exciting field that offers numerous opportunities for research and innovation. From understanding the neural basis of addiction to developing effective interventions for mental health disorders, psychologists continue to make significant contributions to our understanding of human behavior andwell-being.In conclusion, psychology is a vast and complex fieldthat encompasses a wide range of subdisciplines and specialties. From cognitive processes to social interactions, from developmental milestones to neurological disorders, psychology seeks to unravel the mysteries of the human mind and behavior. While it faces numerous challenges and controversies, psychology remains a vital and vibrant science that promises to shed light on some of the most fundamental aspects of what it means to be human.。
英语论文的标题特征
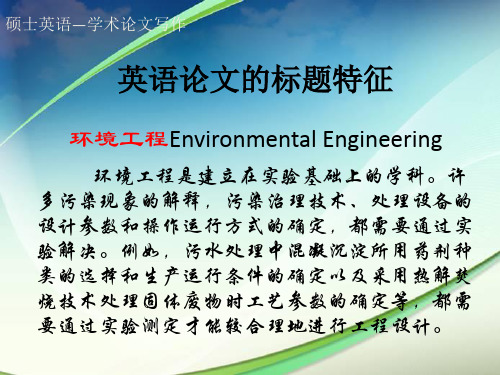
硕士英语—学术论文写作
标题中所用的词尽量使用表达全文内容的关 键词,论文当中的中心词要能够在标题中体 现出来。
1、A parametric study of copper deposition in a fluidized bed electrode
2、Modification of the packed-bed electrode and its potential distribution
3、Electrolytic recovery of metals from waste waters with the Swiss-roll cell
4 、 Electrochemical oxidation of phenol at boron-doped diamond electrode
论文标题中最常出现的是介词,例如of、by、with、in、 on等,每个介词代表的含义不同 1、of频率最高,通常以名词所有格表示逻辑上的主宾关系
硕士英语—学术论文写作
需要探讨的问题:
一、用using连接的标题
1、Adsorption of lead using a new green material
obtained from Portulaca plant
2、Denitrification
of
nitrate-contaminated
groundwater using biodegradable snack ware as
Treatment of Landfill Leachate by Fenton Process with Nano sized Zero Valent Iron particles 2、by和with表示研究的方法和工具
电化学的英语

电化学的英语《Electrochemistry: Understanding the Power of Chemical Reactions》Electrochemistry is a fascinating field of study that concerns the interplay between electrical and chemical phenomena. It deals with the relationships between electricity and chemical reactions, and has a wide range of applications in areas such as energy storage, corrosion prevention, and even medicine.At its core, electrochemistry is based on the concept of redox reactions, or oxidation-reduction reactions. These reactions involve the transfer of electrons between different chemical species, and are at the heart of many important processes in nature and in technology.One of the most widely recognized applications of electrochemistry is in the field of batteries and fuel cells. These devices rely on redox reactions to store and release energy, and are essential for a wide range of everyday technologies, from smartphones and laptops to electric vehicles and renewable energy systems.In addition to energy storage, electrochemistry also plays a crucial role in corrosion prevention and protection. By harnessing the power of redox reactions, scientists and engineers are able to develop coatings and treatments that can protect metal surfaces from corroding, extending the lifespan of infrastructure and machinery.In the realm of medicine, electrochemistry has found applications in fields such as biosensors and drug delivery systems. By leveraging the ability to control and manipulate redox reactions, researchers are able to develop novel technologies for diagnosing diseases and delivering therapeutic agents with unprecedented precision and efficiency.Overall, electrochemistry is a field with a wide range of practical applications and a deep understanding of the fundamental principles at the heart of many important processes. As our world continues to seek sustainable and efficient solutions to global challenges, the study of electrochemistry will undoubtedly play a key role in shaping the future of technology and science.。
Molecular_Catalysis_of_Electrochemical_Reactions._Mechanistic_Aspects
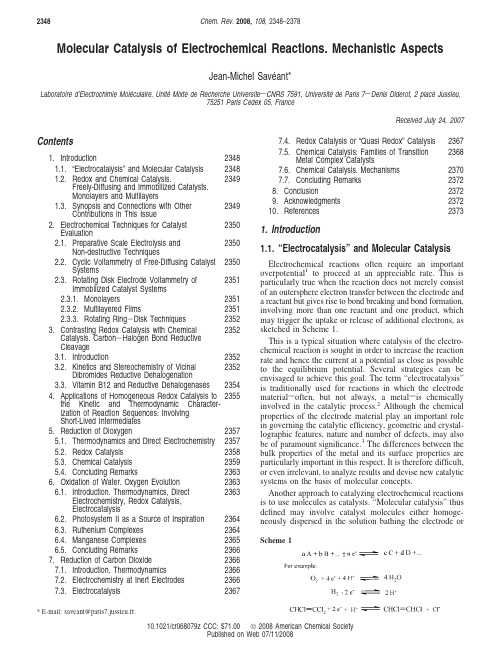
Molecular Catalysis of Electrochemical Reactions.Mechanistic AspectsJean-Michel Savéant*Laboratoire d’Electrochimie Mole´culaire,Unite´Mixte de Recherche Universite s CNRS7591,Universite´de Paris7s Denis Diderot,2place Jussieu,75251Paris Cedex05,FranceReceived July24,2007Contents1.Introduction23481.1.“Electrocatalysis”and Molecular Catalysis23481.2.Redox and Chemical Catalysis.Freely-Diffusing and Immobilized Catalysts.Monolayers and Multilayers23491.3.Synopsis and Connections with OtherContributions in This Issue23492.Electrochemical Techniques for CatalystEvaluation23502.1.Preparative Scale Electrolysis andNon-destructive Techniques23502.2.Cyclic Voltammetry of Free-Diffusing CatalystSystems23502.3.Rotating Disk Electrode Voltammetry ofImmobilized Catalyst Systems23512.3.1.Monolayers23512.3.2.Multilayered Films23512.3.3.Rotating Ring-Disk Techniques23523.Contrasting Redox Catalysis with ChemicalCatalysis.Carbon-Halogen Bond ReductiveCleavage23523.1.Introduction23523.2.Kinetics and Stereochemistry of VicinalDibromides Reductive Dehalogenation23523.3.Vitamin B12and Reductive Dehalogenases23544.Applications of Homogeneous Redox Catalysis tothe Kinetic and Thermodynamic Character-ization of Reaction Sequences:InvolvingShort-Lived Intermediates23555.Reduction of Dioxygen23575.1.Thermodynamics and Direct Electrochemistry23575.2.Redox Catalysis23585.3.Chemical Catalysis23595.4.Concluding Remarks23636.Oxidation of Water.Oxygen Evolution23636.1.Introduction.Thermodynamics,DirectElectrochemistry,Redox Catalysis,Electrocatalysis23636.2.Photosystem II as a Source of Inspiration23646.3.Ruthenium Complexes23646.4.Manganese Complexes23656.5.Concluding Remarks23667.Reduction of Carbon Dioxide23667.1.Introduction.Thermodynamics23667.2.Electrochemistry at Inert Electrodes23667.3.Electrocatalysis23677.4.Redox Catalysis or“Quasi Redox”Catalysis23677.5.Chemical Catalysis:Families of TransitionMetal Complex Catalysts23687.6.Chemical Catalysis.Mechanisms23707.7.Concluding Remarks23728.Conclusion23729.Acknowledgments237210.References2373 1.Introduction1.1.“Electrocatalysis”and Molecular Catalysis Electrochemical reactions often require an important overpotential1to proceed at an appreciable rate.This is particularly true when the reaction does not merely consist of an outersphere electron transfer between the electrode and a reactant but gives rise to bond breaking and bond formation, involving more than one reactant and one product,which may trigger the uptake or release of additional electrons,as sketched in Scheme1.This is a typical situation where catalysis of the electro-chemical reaction is sought in order to increase the reaction rate and hence the current at a potential as close as possible to the equilibrium potential.Several strategies can be envisaged to achieve this goal.The term“electrocatalysis”is traditionally used for reactions in which the electrode material s often,but not always,a metal s is chemically involved in the catalytic process.2Although the chemical properties of the electrode material play an important role in governing the catalytic efficiency,geometric and crystal-lographic features,nature and number of defects,may also be of paramount significance.3The differences between the bulk properties of the metal and its surface properties are particularly important in this respect.It is therefore difficult, or even irrelevant,to analyze results and devise new catalytic systems on the basis of molecular concepts.Another approach to catalyzing electrochemical reactions is to use molecules as catalysts.“Molecular catalysis”thus defined may involve catalyst molecules either homoge-neously dispersed in the solution bathing the electrode or*E-mail:saveant@paris7.jussieu.fr.Scheme1 Chem.Rev.2008,108,2348–2378234810.1021/cr068079z CCC:$71.00 2008American Chemical SocietyPublished on Web07/11/2008immobilized in a monolayer or multilayered coating depos-ited on the electrode surface as sketched in Figure 1.In line with the general theme of this special issue,this review is mostly concerned with molecular catalysis.Nev-ertheless,discussion of examples of electrocatalysis will not be systematically excluded.Analysis of the similarities and differences between electrocatalysis and molecular catalysis of the same global reaction may indeed benefit from the understanding of the mechanism in each case.1.2.Redox and Chemical Catalysis.Freely-Diffusing and Immobilized Catalysts.Monolayers and MultilayersHomogeneous catalysis is concerned with systems in which the catalyst diffuses freely in the solution that contains the substrate.Testing molecular catalysts implies that the electrode material does not participate in the electrochemical reaction.In other words,the electrode plays the role of a heteroge-neous outersphere electron donor (or acceptor).The direct reduction (or oxidation)of the substrate requires a sizable overpotential to give rise to a significant current.The catalyst is usually one member of a reversible couple,P/Q,the standard potential of which is located in between the standard potential of the global electrochemical reaction and the potential where the direct electrochemical reaction occurs.The closer the two standard potentials and the larger the current,the better the catalyst.As exemplified later,catalytic currents may be observed even when the homogeneous electron transfer between the active form of the catalyst is an outersphere reaction.As discussed in the next section,the very existence of “redox catalysis”derives from the fact that,instead of being confined within a two-dimensional space,the electrons to be transferred are then dispersed in a three-dimensional space.“Chemical catalysis”involves moreintimate interactions between the active form of the catalyst and the substrate,thus opening a route to more efficient and more specific catalytic processes.In many,but not all,practical applications immobilization of the catalyst molecules onto the electrode surface,as sketched in Figure 1,is advantageous.Catalysis is expected to be more efficient at multilayered coatings than at mono-layer coatings,provided transport of electrons,substrate and product through the film does not become rate-limiting.It follows from the very nature of redox catalysis that it is possible in multilayered films but not at monolayer coatings,since redox catalysis implies that the electrons to be transferred be dispersed in a three-dimensional space.1.3.Synopsis and Connections with Other Contributions in This IssueSection 2discusses the techniques that may be used to evaluate a catalyst in terms of efficiency and selectivity.Section 3is devoted to the description of a particularly clear example illustrating the distinction between redox and chemical catalysis both in terms of efficiency and specificity.In the fourth section,we review the application of redox catalysis to the kinetic characterization of electron transfer mechanisms involving fast decaying intermediates.Sections 5–7are dedicated to selected examples where chemical catalysis is particularly important.The examples have been selected in relation with the activation of small molecules relevant to the present challenges of renewable energy and greenhouse effects:reduction of oxygen (section 5),oxygen evolution from the oxidation of water (section 6),reduction of carbon dioxide (section 7).The links that may exist with enzymes that catalyze the same reactions will be emphasized.These enzymes have served as sources of inspiration for the catalyst design or,conversely,the chemical catalyst may be used as a simplified model of the enzyme aiming at a better understanding of enzymatic mechanisms.In spite of its paramount importance,we let aside catalysis ofhydrogenFigure 1.Schematic representation of the various types of molecular catalysis of electrochemicalreactions.Jean-Michel Savéant received his education in the Ecole Normale Supérieure in Paris,where he became the Vice-Director of the Chemistry Department before moving to the University Denis Diderot (Paris 7)as a Professor in 1971.He is,since 1985,Directeur de Recherche au Centre National de la Recherche Scientifique in the same university.In 1988-1989he was a distinguished Fairchild Scholar at the California Institute of Technology.His current research interests involve all aspects of molecular and biomolecular electrochemistry as well as mechanisms and reactivity in electron transfer chemistry and biochemistry.Among many distinctions,Jean-Michel Savéant received the Faraday Medal of the Royal Chemical Society,the Olin Palladium Medal of the Electrochemical Society,la Medaglia Luigi Galvani della SocietàChimica Italiana and the Manuel Baizer Award of the Electrochemical Society.Jean-Michel Savéant is a member of the French Academy of Sciences and foreign associate of the National Academy of Sciences of the United States of America.Molecular Catalysis of Electrochemical Reactions Chemical Reviews,2008,Vol.108,No.72349evolution and uptake because it is treated in detail in a preceding Chemical Re V iews thematic issue“Hydrogen”. Numerous other examples,dealing with the organometallic catalysis of important organic reactions,can be found in the contribution of Anny Jutand,to this issue“Contribution of Electrochemistry to Organometallic Catalysis”.Reversing the concept of chemical catalysis of electrochemical reactions leads to the notion of using electrons(or holes)from an electrode to catalyze a chemical reaction.This important topic is treated in the contribution of Abdelaziz Houmam,“Elec-tron Transfer Initiated Reactions:Bond Formation and Bond Dissociation”.Homogeneous and supported catalysis of electrochemical reactions by enzymes are massively present in the contribution of Christophe Le´ger“Direct Electro-chemistry of Redox Enzymes as a Tool for Mechanistic Studies”.2.Electrochemical Techniques for Catalyst Evaluation2.1.Preparative Scale Electrolysis andNon-destructive TechniquesNon-destructive techniques,such as cyclic voltammetry operated with a microelectrode,are particularly useful for a first evaluation of the catalyst:determination of the potential at which the catalytic process can be run and of the catalytic efficiency as measured by the current densityflowing through the electrode at this potential for a given concentration or partial pressure of the substrate.They may then be used by means of a more detailed kinetic analysis to unravel the mechanism of the catalytic reaction and suggest improve-ments.Gauging of the selectivity of the catalytic reaction requires moving to preparative-scale electrolysis and deter-mining the faradaic yields of each of the reaction products. Such sustained electrolyses are also necessary to estimate the stability of the catalyst by observing the variation of the preparative-scale current with time.Following the cyclic voltammetric response simultaneously is an additional way of observing the evolution of the catalyst in the course of electrolysis.Preparative-scale evaluation is thus required to establish the actual performances and viability of catalytic systems beyond the rapid test that cyclic voltammetric allows. 2.2.Cyclic Voltammetry of Free-Diffusing CatalystSystemsCyclic voltammetry has been and still is the most popular non-destructive technique applied to homogeneous catalysis systems,although other techniques might be used as well. Figure2summarizes the various characteristic shapes of the cyclic voltammetric responses expected for a catalytic reaction of the type shown in Figure1,in which the rate-determining step(rate constant k)isfirst order in substrate (bulk concentration:C A)and catalyst(bulk concentration: C P).The responses are governed by two(and only two) dimensionless parameters,taken as coordinates of the“kinetic zone diagram”shown in Figure2.4,5The most familiar situation is that of a large excess of substrate over catalyst(right-hand“no substrate consump-tion”zone)where the cyclic voltammetric response passes from a reversible“no catalysis”response proportional to the square root of the scan rate to an S-shaped curve independent of the scan rate as the catalytic rate constant increases and/or the scan rate decreases.6–8The plateau current density, I p,is then an easy measure of the rate constant(D P:catalyst diffusion coefficient):Ip)FCP√D P√kC AThe“pure kinetic”conditions thus achieved prevail when the catalytic reaction is fast as compared to diffusion,i.e., when the reaction layer within which the concentration profiles of the catalyst P and Q forms are confined is much thinner than the diffusion layer.At the left-hand end of diagram,consumption of the catalyst increasingly interferes up to the“total catalysis”situation where thefirst wave of the two-wave system that develops then is governed by the diffusion of the substrate.The peak current density,I p,Ip)0.609√FC A F V RTis no longer a function of the catalytic rate constant,which instead governs the location of the peak potential:Ep)EP⁄Q0-0.409RTF+RTFln(RT F kC P2C A V)in the cases where the electron transfer between the electrode and the catalyst couple is fast(standard potential:E P/Q0). How the variations of the experimental concentrations and rate parameters induce the passage from one kinetic situation to the other,in direction and magnitude,is summarized by the red compass rose on top of the diagram.More complicated kinetic schemes may be encountered in practice as,for example,when two-electron stoichiom-etries,mixed kinetic control by successive steps,partial deactivation of the catalyst etc.are involved.Several of these schemes are analyzed in ref9.Any homogeneous catalytic mechanism may be analyzed by“digital simulation”10of the cyclic voltammetric responses by means offinite difference resolution of the set of diffusion-reaction partial derivative equations and initial and boundary conditions.11Two main commercial packages,Digisim12and DigiElech13are avail-able for this purpose.In the application of these powerful procedures to reaction schemes involving several successive and/or competing steps,a preliminarily dimensionlessanaly-Figure2.Kinetic zone diagram showing the expected shapes of cyclic voltammetric responses as a function of the two dimension-less parameters taken as coordinates for a catalytic reactionfirst order in substrate(bulk concentration:C A)and catalyst(bulk concentration:C P).V:scan rate.2350Chemical Reviews,2008,Vol.108,No.7Savéantsis of the kinetic problem is advisable so as to determine the minimum number of governing dimensionless param-eters.This is a necessary step for a lucid assignment of the mechanism and a realistic estimate of grouped rate and equilibrium constants.Examples will be given in section 4.2.3.Rotating Disk Electrode Voltammetry of Immobilized Catalyst Systems2.3.1.MonolayersThere are many ways to attach monolayer or multilayered films containing redox centers onto electrode surfaces.14,15While cyclic voltammetry and other transient techniques could be used to investigate such systems,rotating disk electrode voltammetry (RDEV)has been mostly used instead.For monolayer coatings and a catalytic reaction that is first order in substrate and in catalyst,the current density (I )-potential (E )curve is 16I I A)I k I A1+I k I A +exp [F RT(E -E P⁄Q 0)]which depends on a single dimensionless competitionparameter,I k /I A ,itself defined as the ration of two current densities:I k )Fk ΓP C A (ΓP ,total surface concentration of catalyst),which characterizes the catalytic reaction,and I )FC A D A /δ)0.62FC A D A 2/3ν-1/6ω1/2(δ,thickness of the diffusion layer;ν,kinematic viscosity;ω,rotation rate),which characterizes the mass transport of the substrate.The two limiting situations reached for I k /I A small and large are of the same type as the “pure kinetic”and “total catalysis”conditions,respectively,depicted in section 2.2for the cyclic voltammetry of homogeneous catalytic systems.The plateau current density may be expressed as1I p )1Fk ΓP C A +10.62FC A D A2⁄3ν-1⁄6ω1⁄2giving rise to a “Koutecky -Levich”plot of the variation of the inverse of the current density with the inverse of the square root of the rotation rate (Figure 3),from the intercept of which the catalytic rate constant,k ,can be estimated if the surface concentration of the catalyst,ΓP ,is known (if not,k ΓP nevertheless provides an interesting characterization of the catalytic properties of the electrode coating).2.3.2.Multilayered FilmsExpression of the governing parameters by means of characteristic current densities is also worthwhile in the caseof multilayered coatings.17Besides the catalytic reaction and the diffusion of the substrate in the bathing solution that are characterized by the same current densities as above (ΓP is now the total amount of catalyst contained in one unit surface area of film,C A is replaced by κC A ,κbeing the partition coefficient of the substrate between the solution and the film),two other rate-limiting factors have to be taken into account,namely,the diffusion of the substrate through the film and electron hopping between the electrode surface and the redox centers in the film,characterized by the current densities,I S and I e :I S )F κC A D S /d f (d f ,film thickness;D S ,diffusion coefficient of the substrate in the film)and I e )FS Γp D e /d f 2(D e ,equivalent diffusion coefficient for electron hopping 18–22).The competition between these rate-limiting factors is represented by the kinetic zone diagram in Figure 4,which corresponds to the simple mechanism depicted in Figure 1.23–26The symbolic designation of each zone summarizes the nature of the kinetic control of the current,R for the catalytic reaction,S for substrate diffusion through the film,E for electron propagation.SR and ER apply to fast catalytic reactions and mean a combined control under the pure kinetic mode,in which the catalytic reaction and the diffusion of either the substrate or the electrons compensates each other,giving rise to a thin reaction layer,of thickness (√I s ⁄I k )d f and (√I e ⁄I k )d f .The expressions of the current in each zone of the kinetic zone diagram of Figures 4are available from Table 4.1of ref 26.Several more complicated processes have been treated according to the similar ap-proaches.These treatments and the resulting equations are given in the same reference.The analyses and expressions of the current form the bases on which contemporary rationalization of electrochemical sensors is dealt with,as developed for example in refs 27and28.Figure 3.Rotating disk electrode voltammetry of a catalytic reaction first order in substrate and in catalyst at a monolayer coating.“Koutecky -Levich”plot.Figure 4.Kinetic zone diagram characterizing the RDEV plateau currents for the reaction scheme in Figure 1.Full lines:substrate concentration profile.Dashed lines:concentration profile of the reduced form of the catalyst.Adapted with permission from Figure 5.5of ref 26.Copyright 1992J.Wiley and Sons.Molecular Catalysis of Electrochemical Reactions Chemical Reviews,2008,Vol.108,No.723512.3.3.Rotating Ring -Disk TechniquesGenerating a product or an intermediate at a disk electrode and collecting it at a ring electrode that concentrically surrounds the disk is an alternative to cyclic voltammetry.The product or intermediate is generated by fixing the disk potential at an appropriate value and scanning the ring potential so as to obtain its oxidative or reductive signature as a steady-state current -potential curve.This technique has been extensively and successfully applied in the determina-tion of the product,H 2O 2or H 2O in the catalytic reduction of oxygen (see section 5.3).3.Contrasting Redox Catalysis with Chemical Catalysis.Carbon -Halogen Bond Reductive Cleavage 3.1.IntroductionAs opposed to redox catalysis,where the catalyst acts as an outersphere electron transfer agent,chemical catalysis involves more intimate interactions between the active form of the catalyst and the substrate.A more precise picture of what is meant by “more intimate interactions”leads to distinguishing two situations.One simply involves bonded interactions in the electron transfer transition state.In other words,electron transfer then possesses an inner-sphere character,which is expected to result in a lower activation energy than for an outersphere electron transfer of same driving force.More stereospecificity is also expected to result from bonded interactions in the transition state.The reduction of vicinal dibromides into the corresponding olefins offers a particularly clear example of this situation both in terms of kinetics and of stereochemistry.Another situation is when the “more intimate interactions”are so strong that an adduct is formed between the active form of the catalyst and the substrate.The potential energy profiles corresponding to the two situations are sketched in Figure 5.The formation of this intermediate is again expected to be faster than an outersphere electron transfer reaction;the more so the more stable the adduct.A problem that may then arise is the rate of decomposition of the adduct that ensures the formation of the products and the regeneration of the catalyst.Catalysis of the reduction of organic halides by vitamin B12offers an illustration of this type of chemical catalysis,in relation with the mechanism by which reductive dehalogenases reduce dangerous polyhalide pollutants.We will focus on these two examples taken in the literature on organic halide reduction,noting that several other importantreactions pertaining to the same topic are discussed in Anny Jutand’s contribution to this issue.3.2.Kinetics and Stereochemistry of Vicinal Dibromides Reductive DehalogenationAn early investigation of the direct electrochemical reduction of these compounds in an aprotic medium (DMF)showed that the corresponding olefin is formed according to a two-electron stoichiometry (overall reaction in Scheme 2).29Further studies 30–37revealed that the transfers of the two electrons are not concerted,although the debrominated radical formed in the first step of Scheme 2is reduced in the second step at a less negative potential than the potential at which the first reaction takes place.The current -potential responses obtained in,e.g.,cyclic voltammetry (Figure 6)thus correspond to a two-electron stoichiometry,even though the two electrons come into the molecule successively.Each of the two successive electron transfers is coupled withtheFigure 5.Redox and chemical catalysis.Potential energy profiles.Scheme2Figure 6.Redox and chemical homogeneous catalysis of trans -1,2-dibromocyclohexane.(a)Cyclic voltammetry in dimethylfor-mamide of the direct electrochemical reduction at a glassy carbon electrode (top),of redox catalysis by fluorenone (middle),of chemical catalysis by an iron(I)porphyrin.(b)Catalysis rate constant as a function of the standard potential of the catalyst couple:green dots,aromatic anion radicals;downward brown triangles,Fe(I);upward red triangles,Fe(0);blue squares,Co(I);magenta dots,Ni(I)porphyrins.Adapted with permission from Figures 3and 4of ref 36.Copyright 1990American Chemical Society.2352Chemical Reviews,2008,Vol.108,No.7Savéantcleavage of a C -Br bond,which occurs concertedly with electron transfer.34,36Since both steps are irreversible and the second step is energetically much easier than the first,the overall two-electron process is kinetically controlled by the first step.The reaction is also an example of the role of conformations in electron transfer,33in this instance,dis-sociative electron transfer.There is a clear antiperiplanar preference for the reaction (as illustrated in Scheme 3in the case of dibromocyclohexane)due to the stabilization of the radical by coupling of the unpaired electron with bromine in the first case.The weaker bond dissociation energy leads to a more favorable standard potential and a weaker intrinsic barrier.When the two conformers are present and can convert one into the other,the reduction follows a “CE”mechanism,which goes through the more reducible of the two.31,33,34,36Since the direct electrochemical reaction is irreversible,it should be possible to catalyze it by redox as well by chemical catalysts.Anion radicals of aromatic molecules are classical homogeneous redox catalysts in aprotic media.Catalytic currents are indeed observed when a dibromoalkane is added to a solution containing the aromatic anion radical parent (see the illustrative example of fluorenone in Figure 6).36The rate constant of the rate-determining step can be derived from the catalytic increase of the catalyst wave (from the black to the green curve in the fluorenone diagram of Figure 6).The resulting variations of the electron transfer rate constant with the driving force,measured by the standard potential of the catalyst,are shown in the right-hand diagram of Figure 6(green dots)in the case of trans -1,2-dibromocy-clohexane.They are in quantitative agreement with the dissociative electron transfer kinetics predicted on the basis of the electrochemical results.38Stereochemical studies carried out with meso and d ,l 4,5-dibromooctane showed partial stereoselectivity in the formation of the E and Z olefin (Scheme 4),thus suggesting the mechanism depicted in thesame scheme,where the E /Z interconversion at the level of the monobromo radical resulting from the first dissociative electron transfer competes with the second electron transfer,leading to an E :Z ratio different from thermodynamic equilibrium.The redox nature of catalysis is thus attested both by the lack of stereospecificity of the reaction and by the kinetic constraint deriving from the outersphere character of the electron donor.The corresponding activation free energy is indeed given by∆G q )(λ⁄4)[1+F (E catalyst 0-E substrate)⁄λ]2where E substrate 0is the standard potential of the reaction being catalyzed and λthe total reorganization energy that includes,in particular the dissociation of the bond being cleaved concertedly with electron transfer.39This equation corre-sponds to the green line in the right-hand diagram of Figure 6.Catalysis of the reduction of trans -1,2-dibromocyclohex-ane by various Fe(I),Fe(0),Co(I),Ni(I)porphyrins is also represented in Figure 6.Similar results were obtained for other 1,2-dibromides.36It clearly appears that,for the same driving force,the rate constants for these catalysts are systematically larger than for the redox catalysts.This kinetic advantage,which reaches several orders of magnitude,points to the conclusion that these various low-valent metallopor-phyrins behave as chemical catalysts .This conclusion is confirmed by the observation that the reaction is now completely stereoselective,pointing to the mechanism shown in Scheme 5.The Br +transfer to the metallic center is followed by an immediate expulsion of the second bromine under the form of Br -,which is faster than E /Z intercon-version or even concerted with the first step.The same conclusion most likely applies to Co(I)vitamin B12(Scheme 7),which was shown to react fast (k ≈107M -1s -1)in an aprotic medium at a potential of only -0.5V vs SHE.40The reduction of vicinal dihalides has been used as a test reaction to evaluate the catalytic properties of vitamin B12in microemulsions,in films deposited on the electrode surface,41–49as well as in biphasic systems with vitamin B12in water and dibromocyclohexane in oil.50,51The observations made with the complexes shown in Scheme 6as catalysts of the reduction of trans -1,2-dibromocyclohexane are also relevant to the distinction between redox and chemical catalysis.As seen earlier,nickel tetraphenylporphyrin (TPPNi)gives rise to chemical cataly-Scheme3Scheme4Scheme5Molecular Catalysis of Electrochemical Reactions Chemical Reviews,2008,Vol.108,No.72353。
electrochemic acta 电催化 -回复

electrochemic acta 电催化-回复什么是电催化?电催化是一种利用电化学方法促进化学反应的技术。
通过在电极表面加入电化学催化剂,电荷的传递可以加速化学反应的进行,从而改善反应活性、选择性和效率。
电催化技术在能源转换、环境保护、化学合成等领域具有广泛的应用。
电催化反应的基本原理是利用外加电位来控制电子的传递。
在电极表面,催化剂接收电子,并将这些电子用于与溶液中的物质发生反应。
电催化反应可以通过改变电极电位来控制反应速率,使其达到正常热动力学条件下无法实现的高速率。
电催化技术存在一定的优势,例如能够在较低温度下进行反应,具有高效性和选择性。
此外,电催化过程中产生的物质可以直接利用或进一步转化,具有较高的化学价值。
电催化技术在能源转换领域有重要应用。
目前,传统能源资源的储备和使用面临着日益严重的问题。
电催化技术可以通过利用可再生能源,例如太阳能和风能,在低温下进行电化学反应,来转化成更高能量状态的物质,例如氢气或高能量电池。
这种能源转换方式不仅高效、环保,而且具有巨大的潜力。
在环境保护领域,电催化技术也可以用于处理废水和废气。
通过外加电位和添加适当的催化剂,可以促使有害物质的氧化还原反应发生,转化成无毒或低毒的物质。
电催化反应不仅可以高效去除污染物,还可以选择性地将有害物质转化为有价值的化合物,从而实现资源的利用。
此外,电催化技术还可以用于化学合成过程中的有机合成反应。
含有自由基的反应通常需要高温和环境有机溶剂,但这种条件不利于反应选择性和产率的提高。
电催化技术可以通过调控电极表面的催化活性位点和电位,改变反应路径,从而实现高选择性、高产率的有机合成反应。
总的来说,电催化技术在能源转换、环境保护和化学合成等领域具有重要应用价值。
随着电催化理论和催化剂设计的不断发展,相信电催化技术将会在未来发挥更加重要的作用。
正常成年CBA小鼠短声诱发听性脑干反应测试波形分析
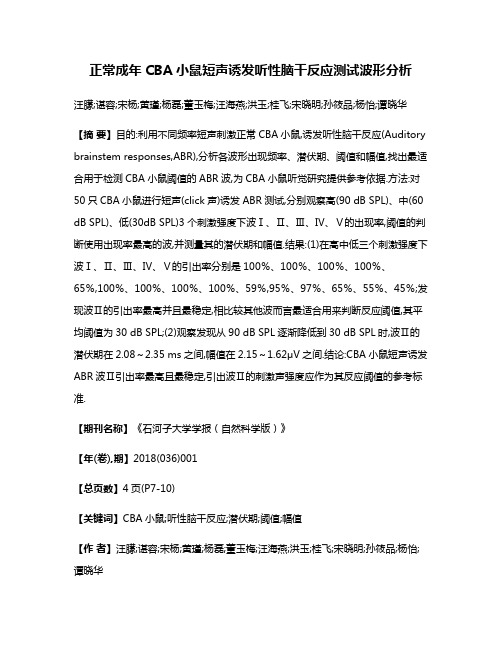
正常成年CBA小鼠短声诱发听性脑干反应测试波形分析汪朦;谌容;宋杨;黄瑾;杨磊;董玉梅;汪海燕;洪玉;桂飞;宋晓明;孙筱品;杨怡;谭晓华【摘要】目的:利用不同频率短声刺激正常CBA小鼠,诱发听性脑干反应(Auditory brainstem responses,ABR),分析各波形出现频率、潜伏期、阈值和幅值,找出最适合用于检测CBA小鼠阈值的ABR波,为CBA小鼠听觉研究提供参考依据.方法:对50只CBA小鼠进行短声(click声)诱发ABR测试,分别观察高(90 dB SPL)、中(60 dB SPL)、低(30dB SPL)3个刺激强度下波Ⅰ、Ⅱ、Ⅲ、Ⅳ、Ⅴ的出现率,阈值的判断使用出现率最高的波,并测量其的潜伏期和幅值.结果:(1)在高中低三个刺激强度下波Ⅰ、Ⅱ、Ⅲ、Ⅳ、Ⅴ的引出率分别是100%、100%、100%、100%、65%,100%、100%、100%、100%、59%,95%、97%、65%、55%、45%;发现波Ⅱ的引出率最高并且最稳定,相比较其他波而言最适合用来判断反应阈值,其平均阈值为30 dB SPL;(2)观察发现从90 dB SPL逐渐降低到30 dB SPL时,波Ⅱ的潜伏期在2.08~2.35 ms之间,幅值在2.15~1.62μV之间.结论:CBA小鼠短声诱发ABR波Ⅱ引出率最高且最稳定,引出波Ⅱ的刺激声强度应作为其反应阈值的参考标准.【期刊名称】《石河子大学学报(自然科学版)》【年(卷),期】2018(036)001【总页数】4页(P7-10)【关键词】CBA小鼠;听性脑干反应;潜伏期;阈值;幅值【作者】汪朦;谌容;宋杨;黄瑾;杨磊;董玉梅;汪海燕;洪玉;桂飞;宋晓明;孙筱品;杨怡;谭晓华【作者单位】石河子大学医学院,新疆石河子832002;杭州师范大学医学院,浙江杭州310036;杭州师范大学医学院,浙江杭州310036;石河子大学医学院,新疆石河子832002;杭州师范大学医学院,浙江杭州310036;石河子大学医学院,新疆石河子832002;石河子大学医学院,新疆石河子832002;杭州师范大学医学院,浙江杭州310036;杭州师范大学医学院,浙江杭州310036;杭州师范大学医学院,浙江杭州310036;石河子大学医学院,新疆石河子832002;杭州师范大学医学院,浙江杭州310036;杭州师范大学医学院,浙江杭州310036【正文语种】中文【中图分类】R764.4随着社会、经济的发展,噪声暴露的人群范围不断扩大。
- 1、下载文档前请自行甄别文档内容的完整性,平台不提供额外的编辑、内容补充、找答案等附加服务。
- 2、"仅部分预览"的文档,不可在线预览部分如存在完整性等问题,可反馈申请退款(可完整预览的文档不适用该条件!)。
- 3、如文档侵犯您的权益,请联系客服反馈,我们会尽快为您处理(人工客服工作时间:9:00-18:30)。
Electrophysiological Correlates of Recollecting Faces of Known andUnknown IndividualsKen A.Paller,Brian Gonsalves,Marcia Grabowecky,Vladimir S.Bozic,and Shishin Yamada Department of Psychology,Northwestern University,Evanston,Illinois60208-2710Received March23,1999We recorded brain potentials from healthy human subjects during a recognition test in order to monitor neural processing associated with face recollection. Subjectsfirst attempted to memorize40faces;half were accompanied by a voice simulating that person speaking(e.g.,‘‘I’m Jimmy and I was a roadie for the Grateful Dead’’)and half were presented in silence.In the test phase,subjects attempted to discriminate both types of old faces(i.e.,‘‘named’’and‘‘unnamed’’faces) from new faces.Recognition averaged87%correct for named faces,74%correct for unnamed faces,and91% correct for new faces.Potentials to old faces were more positive than those to new faces from300to600ms after face onset.For named faces,the old–new ERP difference was observed at anterior and posterior scalp locations.For unnamed faces,the old–new ERP difference was observed only at posterior scalp loca-tions.Results from a prior experiment suggest that these effects do not reflect perceptual priming of faces. The posterior portion of the old–new ERP difference was thus interpreted as a neural correlate of retrieval of visual face information and the anterior portion as an indication of retrieval of person-specific semantic information.2000Academic PressINTRODUCTIONA face can function as an effective memory cue, provoking the retrieval of a wealth of stored informa-tion about an individual.Yet,the brain events that allow us to remember the people we know are largely unknown.Neuropsychological studies of patients with brain damage suggest that perceiving and remember-ing faces depend on processing in specific cortical regions.Additional information about the relevant physiological mechanisms may be revealed by measur-ing brain activity during normal face processing.Here we show that measures of the electrical activity of the brain can be used toward this end.Person recognition—defined as remembering a known individual and retrieving an assemblage of person-specific information pertaining to that individual—generally begins with the perceptual processing of a facial image.Voice information,contextual cues,expec-tations,inferences,and other factors often combine to allow a person to be recognized,but the facial image in isolation can be sufficient for person recognition.The number of distinct faces that an individual can accu-rately recognize is exceedingly large.People become experts at recognizing faces through extensive practice over years and perhaps by virtue of specially evolved brain mechanisms(Carey,1992).Clues about the rel-evant neural mechanisms have been provided by behav-ioral studies in patients and in healthy individuals, single-unit neurophysiology in monkeys,and neuroim-aging and electrophysiological studies in humans(for recent reviews,see De Renzi,1997;Farah et al.,1998; Young,1998).Evidence from these various sources can be interpreted within the context of the theoretical framework for face recognitionfirst put forth by Bruce and Young(1986).Separate modules were postulated for processing physical features of a face,for determin-ing that a face is familiar,for retrieving stored informa-tion about a person,for retrieving a name associated with a face,for expression analysis,and for facial speech analysis.Cortical processing mechanisms specialized for face recognition have been investigated with a variety of methods.In monkeys,particular neurons in temporal cortex respond selectively to faces(Desimone,1991; Perrett et al.,1992).In humans,recordings from intra-cranial electrodes have demonstrated face-specific re-sponses from small regions of the left and right fusi-form and inferior temporal gyri,and electrical stimulation from these same electrodes frequently dis-rupted naming of faces(Allison et al.,1994a).Record-ings from scalp electrodes have also revealed potentials thought to be relatively face-specific(Bentin et al., 1996;Bo¨tzel and Gru¨sser,1989;Jeffreys,1989;Jeffreys et al.,1992).These event-related potentials or ERPs generally appear150to200ms after the onset of a face and have been labeled N170potentials,denoting their negative polarity and170-ms peak latency.N170andNeuroImage11,98–110(2000)doi:10.1006/nimg.1999.0521,available online at on98other similar potentials have been related not only to face-specific processing,but also to eye-gaze-specific processing,and they are thought to reflect cortical activity in occipitotemporal and posterior fusiform re-gions(Allison et al.,1994b;Puce et al.,1996,1997). Functional activation of these same cortical regions has also been associated with face processing using magne-toencephalography or MEG(Linkenkaer-Hansen et al., 1998;Sams et al.,1997),positron emission tomography or PET(Haxby et al.,1996;Sergent et al.,1992),and functional magnetic resonance imaging or FMRI(Clark et al.,1996;Haxby et al.,1999;Kanwisher et al.,1997; Puce et al.,1995).Although the ability to perceptually analyze faces is generally thought to be separate from the ability to remember faces(e.g.,Carlesimo and Caltagirone,1995), it is reasonable to speculate that the inferior occipito-temporal regions where face-specific responses are produced may also be critical for remembering faces. Accordingly,person recognition may depend on interac-tions between these cortical regions and regions that store information pertaining to person identity.In other words,the complex recollective experience that can be cued by a face may depend on a network of stored associations between a visual representation of that face and other information such as person-specific biographical details,sets of relevant episodic memo-ries,emotional associations,and so on.In the present experiment,we investigated scalp-recorded brain potentials that occur when subjects engage in person recognition in response to viewing facial images.Prior investigations of ERPs and memory have usually used words instead of faces(for reviews, see Johnson,1995;Paller,1993,in press;Rugg,1995).A pervasivefinding in this literature is that late positive ERP amplitudes tend to be greater for repeated items compared to new items,sometimes referred to as an old–new ERP difference or ERP repetition effect.Like-wise,in experiments with faces,a repeat presentation of a face generally yields a different ERP response compared to the initial response to that face(Barrett et al.,1988;Begleiter et al.,1995;Bentin and McCarthy, 1994;Bentin and Moscovitch,1988;Hertz et al.,1994; Mu¨nte et al.,1997,1998;Potter and Parker,1997; Schweinberger et al.,1995;Smith and Halgren,1987; Sommer et al.,1997;Uhl et al.,1990).For example, Mu¨nte and colleagues(1997)studied ERPs elicited by faces in implicit and explicit memory tests.In the implicit test,subjects were required to detect famous faces interspersed in a series of nonfamous faces,some of which repeated.In the explicit test,subjects were required to discriminate previously seen faces from other,new faces.In both tests,ERP differences associ-ated with face repetition took the form of increased positivity from about300to700ms.ERP differences were generally smaller in the implicit test than in the explicit test,with additional topographic differences in the300to500ms interval.Presumably,subjects given the implicit memory test engaged in less recollective processing in response to repeated faces than did those given the explicit memory test.Paller and colleagues(1999)recorded ERPs to previ-ously seen faces and new faces,and in addition,two types of previously seen faces were compared—some faces were associated with brief biographical informa-tion in a study phase and some were not.Subjects were instructed to remember the faces with biographies and to forget the others.Despite the artificial nature of these circumstances,a face associated with a biography in this manner can be thought of as corresponding to the face of a known individual.When those known faces were presented in the experiment,recollective process-ing of the sort typically associated with person recogni-tion was presumably engaged.ERPs elicited by those faces included an enhanced response over posterior scalp regions and,to a lesser extent,over anterior regions.The present study was designed to determine whether similar ERP correlates of face recollection can also be observed during a yes–no recognition test and without differential instructions to remember.The experiment was thus arranged so that a direct comparison could be made between faces associated with biographical infor-mation and faces presented under circumstances that were identical except for the absence of associated biographical information.As a shorthand,we will refer to the former category of faces as named faces and to the latter category of faces as unnamed faces.This contrast corresponds to the real-world contrast be-tween faces of known and unknown individuals.Faces of both types can potentially provoke recollection,as someone can remember having seen a face before regardless of whether any person-specific biographical information is known.We hypothesized that ERP rep-etition effects would be found for named faces and,to a lesser extent,for unnamed faces,which may in both cases reflect recollective processing.METHODSSubjectsA group of four men and eight women participated in the experiment.The mean age was20.6years(range18 to26years).All subjects were right-handed by self-report.Subjects gave informed consent and were paid for their participation.StimuliVisual stimuli included photographs of180faces from a1970s high school yearbook.Each face was presented in grayscale within a rectangular area99MONITORING FACE RECOLLECTIONmeasuring 12.5by 16cm in the center of a computer screen.Faces were viewed from a distance of 135cm (such that the rectangular stimuli subtended 5.9by 7.5°visual angle).A set of 40faces was used in the study phase.These faces were shown again in the test phase along with 80new faces.Another 60new faces were used in a paper-and-pencil recognition test.Each set of faces included an equal number of women and men.Auditory stimuli were paired with 20of the faces in the study phase.These stimuli were spoken by 10women and 10men so as to simulate the experience of meeting the people depicted.Each voice included a name and some brief biographical information (see Paller et al.,1999,for additional details).ProcedureThe procedure included a study phase followed imme-diately by a test phase and then a paper-and-pencil recognition test.Each subject was tested individually.To reduce artifactual contamination of EEG recordings,subjects were instructed to minimize muscle tension,eye movements,and blinks during experimental runs.During the study phase (Fig.1A),subjects were instructed to try to remember a series of people.They were told that 20faces (named faces)would be pre-sented with a spoken introduction to approximate the experience of actually meeting these people and that 20faces (unnamed faces)would be presented in silence.Subjects were advised to try to remember all of the people for a memory test that would be given later.They were told that the test would assess their ability to recognize the faces and to recall the names and biographical information of the people who spoke.Faces were shown for 300ms at a rate of 1every 5s.The onset of the voice for each named face coincided with the onset of the face presentation.The entire setofFIG.1.Schematic representation of experimental trials.(A)The study phase included faces with voices (named faces)and faces without voices (unnamed faces).(B)The test phase included faces from the study phase and new faces,with no auditory components.100PALLER ET AL.40faces was presented three times using different random orders.The sets of faces assigned to named and unnamed conditions were counterbalanced across sub-jects.In other words,each face stimulus from the set served as a named face for six subjects and as an unnamed face for the other six subjects.During the test phase(Fig.1B),subjects were in-structed to respond after each face according to whether the face shown was old or new,pressing a button in one hand or the other(right hand for old for half of the subjects,left hand for old for the others).They were also told to use this as an opportunity to think about the biographical information that was to be remembered for the subsequent memory test.Faces were shown for 300ms at a rate of1every3s.Faces were presented in four runs without any auditory d and unnamed faces were repeated across runs,whereas new faces each appeared on only one occasion.Because we wished to make comparisons with results from a prior experiment,we used stimulus sequences identical to those used previously,even though the task was changed.In the prior experiment(Paller et al.,1999), target events were included by selecting2new faces,2 named faces,and2unnamed faces in each run to be presented twice in a row.In the present experiment, subjects were told that when a face appeared twice in a row,they should respond the same way for both presen-tations.Responses to these immediately repeated faces were excluded from all analyses.Thus,the remaining faces in each of the four runs included a randomly ordered set of60faces:the20named faces,the20 unnamed faces,and the20new faces.For the paper-and-pencil recognition test given at the conclusion of the experiment,subjects used a set offive pages showing20faces per page.These100faces included the40faces from the study phase randomly mixed with60new faces not otherwise used in the experiment.Subjects were asked to place a letter in a corresponding box for each of the20named faces and each of the20unnamed faces and to write down the name and biographical information for each named face.Approximate wording was sufficient for recalled biographical information to be scored as correct.Electrophysiology Electroencephalographic recordings were made from 21scalp electrodes embedded in an elastic cap at standard locations(Fpz,Fz,Cz,Pz,Oz,Fp1,Fp2,F3, F4,F7,F8,C3,C4,P3,P4,O1,O2,T3,T4,T5,T6).A left mastoid reference electrode was used online and the reference was changed offline to the average of left and right mastoid recordings.Two channels were used for monitoring horizontal and vertical eye movements and trials contaminated by electroocular artifacts were excluded from the analyses(7.2%on average in the test phase).Biosignals were amplified with a0.1to100Hz band pass and sampled at a rate of250Hz.ERPs were computed for1024-ms epochs beginning100ms prior to stimulus onset.The300-to600-ms interval was se-lected for initial analyses as this interval was used in our earlier study(Paller et al.,1999).Subsequent analyses to investigate ERP time course were con-ducted over100-ms latency intervals.ERP measure-ments were evaluated using analysis of variance (ANOVA),and critical F ratios were based on degrees of freedom adjusted according to the Huynh–Feldt proce-dure when needed to control for Type I errors in repeated-measures designs.RESULTSBehavioral results are summarized in Table1.As expected,recognition was better for named faces than for unnamed faces.This difference was significant for both the test-phase recognition test[t(11)ϭ3.6,Pϭ0.004]and the subsequent paper-and-pencil recogni-tion test[t(11)ϭ 2.5,Pϭ0.03].For named faces, successful name recall averaged33.8%(SEϭ7.3)and successful recall of the other biographical information averaged60.0%(SEϭ5.6).Reaction time results for correct trials in the test phase(Table1)showed that responses were equiva-lently fast for named faces and unnamed faces[t(11)Ͻ1].Responses to both types of old faces were faster than responses to new faces[t(11)ϭ3.5,Pϭ0.01and t(11)ϭ2.3,Pϭ0.04,respectively].Electrophysiological recordings during the test phase revealed systematic differences as a function of condi-tion,as shown in Fig.2.First,note that ERPs computed across all trials were quite similar to ERPs computed for correct trials.However,there was insufficient statis-tical power to analyze ERPs separately for incorrect trials.Given our concern with identifying neural corre-lates of accurate retrieval,ERP results for correct trials will be emphasized.Analyses of the two study ef-fects—(1)ERPs to named versus new faces and(2) ERPs to unnamed versus new faces—will be describedTABLE1Behavioral Results from Memory Tests(SE Shownin Parentheses)MeasureConditionNamed faces Unnamed faces New facesTest-phase recognitionaccuracy(%correct)87.2(3.0)73.9(5.2)90.9(2.6) Paper-and-pencil rec-ognition accuracy(%correct)73.3(4.7)64.2(4.6)89.3(1.8) Mean reaction time(ms)770(30)784(36)848(26)101MONITORING FACE RECOLLECTIONin turn.Both of these study effects (or old–new ERP differences)can also be viewed as difference waves (Fig.3).Mean ERP amplitudes from the five midline scalp locations were initially measured over the interval from 300to 600ms and these measurements were submitted to ANOVAs with Condition (named vs new or unnamed vs new)and Location as factors (see Table 2).The first ANOVA showed that ERPs were significantly more positive for named than for new faces.The Condition by Location interaction reflected the finding that this study effect was significant at all midline locations except the most anterior one.ERP compari-sons between unnamed and new faces revealed a similar pattern.Midline ERPs from 300to 600ms were also significantly more positive for unnamed than for new faces.The Condition by Location interaction re-flected the finding that this study effect was significant only at the two most posterior locations.In other words,study effects at posterior locations (Pz and Oz)were reliable for named and unnamed faces,whereas study effects at anterior locations (Fz and Cz)were reliable only for named faces.The topography of the two study effects can be viewed as a series of interpolated maps created from ERP difference measurements over con-secutive 100-ms intervals (Figs.4and 5).Subsequent analyses were conducted to determine the time course of the study effects by analyzing ERPs over 100-ms intervals.First,analyses focused on re-sults from the midline parietal location,where study effect amplitudes were largest.ERPs to named faces were significantly more positive than ERPs to new faces for all three intervals from 300to 600ms [F (1,11)ϭ8.42,10.61,and 11.85,P ϭ0.01,0.008,and 0.006,respectively].Differences were nonsignificant for all other intervals,although there was a marginal differ-ence from 200to 300ms [F (1,11)ϭ3.8,P ϭ0.08].ERPs to unnamed faces were also significantly more positive than ERPs to new faces for all three intervals from 300to 600ms [F (1,11)ϭ10.84,19.02,and 12.17,P ϭ0.007,0.001,and 0.005,respectively]and nonsignificant for all other intervals.Additional analyses were conducted at the midline frontal location,where only the named–new ERP difference was significant.This difference appeared to begin later than the posterior difference,as it was nonsignificant from 300to 400ms [F (1,11)ϭ2.68,P ϭ0.13],significant from 400to 500ms and from 500to 600ms [F (1,11)ϭ14.00and 6.61,P ϭ0.003andFIG.2.ERPs recorded during the test phase for named faces,unnamed faces,and new faces.Recordings shown were from midline scalp locations arranged from anterior (top)to posterior (bottom),including all trials (left)or only correct trials (right).102PALLER ET AL.0.03,respectively],and nonsignificant for all other intervals.In short,the posterior difference was present from 300to 600ms,whereas the anterior difference was present from 400to 600ms.To directly assess the reliability of anterior ERP differences between named and unnamed faces,ERPs were analyzed over consecutive 100-ms intervals at midline electrodes.For the 400-to 500-ms interval,the difference was significant at Fpz [F (1,11)ϭ6.49,P ϭ0.03],marginal at Fz [F (1,11)ϭ4.09,P ϭ0.07],and nonsignificant at Cz,Pz,and Oz [F (1,11)Ͻ1].For other intervals differences were nonsignificant.In addition,ERP differences from 400to 500ms from all lateral locations were submitted to a Condition by Hemisphere by Location ANOVA.Although there was a tendency for ERP differences between named and unnamed faces to be larger over the right hemisphere (Fig.3),all effects involving Hemisphere were nonsignificant.DISCUSSIONThe study-phase manipulation in the present experi-ment provided richer encoding for named faces than for unnamed faces.The contrast between named and un-named faces was associated with two differences in later memory.First,named faces tended to provoke the retrieval of stored biographical information from the study phase,whereas this was not possible for un-TABLE 2ERP Differences between ConditionsComparison µV SE FPNamed–new faces Midline mean 1.760.51Main effect of Condition*11.920.005Condition ϫLocation interaction *4.680.02Midline locations Fpz 0.790.44 3.210.10Fz 1.500.50*9.100.01Cz 1.930.67*8.200.02Pz 2.640.76*12.060.005Oz1.950.48*16.820.002Unnamed–new faces Midline mean 1.330.45Main effect of Condition*8.880.01Condition ϫLocation interaction *6.970.001Midline Locations Fpz 0.230.430.280.61Fz 0.870.57 2.380.15Cz 1.310.64 4.150.07Pz 2.330.58*16.260.002Oz1.920.37*26.380.0003Note.An asterisk adjacent to the F value indicates a statistically significant effect.Degrees of freedom were 4,44for each Condition ϫLocation interaction and 1,11for all othertests.FIG.3.ERP difference waves computed by subtracting ERPs to new faces from ERPs to old faces,including only correct trials.Recordings shown were from all scalp electrodes,arranged topographically.103MONITORING FACE RECOLLECTION104PALLER ET AL.FIG.4.Topographic maps of ERP differences across the scalp for ERPs to named faces minus ERPs to new faces.A surface spline interpolation was applied to data obtained from each electrode location(indicated by small circles on each schematic view of a head as viewed from above).Maps represent mean amplitude differences computed for consecutive100-ms intervals starting at0and ending at800ms.105MONITORING FACE RECOLLECTIONFIG.5.Topographic maps of ERP differences between unnamed faces and new faces.Topographic maps were created as in Fig.4.named faces.Second,superior recognition performance was observed for named compared to unnamed faces. Subjects recognized named faces more accurately,but reaction times for correct responses did not differ between named and unnamed faces.Furthermore, reaction time distributions for named and unnamed faces appeared extremely similar.Thus,ERP compari-sons for named versus unnamed faces in the test phase were not complicated by confounding differences in reaction time,nor were there any systematic physical stimulus differences(by virtue of the counterbalanced experimental design).Although significant old–new ERP differences were present for both types of old faces, for named faces these effects were found at anterior and posterior scalp locations(Fig.4),whereas for unnamed faces these effects were restricted to posterior scalp locations(Fig.5).In prior experiments conducted with the same named and unnamed faces,subjects were instructed in the study phase to remember the named faces and to forget the unnamed faces(Paller et al.,1999).In a test phase in which perceptually degraded faces were presented for fame decisions(i.e.,‘‘famous’’vs‘‘nonfamous’’), priming was measured as a facilitation in both decision accuracy and latency(Paller et al.,1999,Experiment 1).Importantly,the magnitude of priming did not differ between the two types of studied faces(referred to as remember faces and forget faces).Indeed,differential processing induced by remember versus forget instruc-tions at study generally affects later recall and recogni-tion but does not affect priming performance(Basden et al.,1993;Golding and MacLeod,1998;Johnson,1994; Paller,1990;Roediger and McDermott,1993).The high degree of similarity between procedures used in our prior ERP experiment(Paller et al.,1999,Experiment 2)and in the present experiment allows for straightfor-ward cross-experiment comparisons.The only two ways in which the design of the present experiment differed were that(1)all faces shown in the study phase were to be remembered and(2)the task in the test phase was to make overt recognition responses.Accordingly,it is reasonable to speculate that priming would likewise be matched between named and unnamed faces in the present experiment.In contrast,recognition was supe-rior for named compared to unnamed faces whether or not directed forgetting instructions were included. ERPs elicited in the test phase in the prior ERP experiment(Paller et al.,1999,Experiment2)were found to differ between remember and forget faces. These effects were interpreted in light of the behavioral results that the study-phase manipulation influenced recognition but not priming.Note that no overt recogni-tion responses were made when ERPs were recorded. Subjects were instructed to retrieve learned biographi-cal information when shown a remember face but to make no overt response for either remember faces or forget faces.Recollection,but not priming,thus differed between remember and forget faces.Electrophysiologi-cal correlates of priming would thus be absent in comparisons between the two types of old faces(though ERP correlates of priming have been observed with words,e.g.,Paller and Gross,1998).ERP differences between the two types of old faces(remember faces–forget faces)were thus interpreted as electrophysiologi-cal correlates of recollective processing,divorced from the influence of priming and other nonspecific factors. Figure6shows the spatiotemporal pattern of these effects.Inspection of Figs.4,5,and6suggests that similar neurophysiological phenomena were recorded across experiments,although there were differences in the latency of the observed response.Apparently,these electrophysiological manifestations of face recollection were evident whether or not subjects made overt recog-nition responses and whether or not faces were associ-ated with biographical information.Interestingly,the latency of old–new effects was shorter in the present experiment than in the prior experiment.A reasonable explanation for this difference is that retrieval process-ing occurred more quickly following face presentations in the present experiment due to the recognition task requirement,which demanded a quick manual re-sponse to each face.The comparison across experi-ments is also relevant for several other reasons.Old–new ERP effects in the present experiment could have been influenced by response-related processing,given that reaction times differed between old and new faces. However,the old–new ERP differences cannot be ex-plained by a relative increase in temporal variability of processing elicited by new face presentations,because reaction time variability was less for new faces com-pared to old faces,as shown in Table1.The contrast between named–new and unnamed–new ERP differences in the present experiment makes it possible to ask whether a portion of the effect is specifically related to retrieval of biographical informa-tion.Again,note that this named/unnamed contrast is free from any physical stimulus effects(due to counter-balancing)and from response factors(given the ab-sence of corresponding reaction time differences).If one were to assume that nearly all of the old–new ERP effect reflected biographical retrieval,then the effect would be expected to be much larger for named faces than for unnamed faces.On the contrary,if one were to assume that nearly all of the old–new ERP effect reflected face retrieval,then the effect would be ex-pected to be the same for named and unnamed faces. Indeed,at posterior locations the old–new ERP effect was highly similar for named and unnamed faces.This posterior portion of the effect can thus be taken as an index of face recollection associated with visual process-ing of the facial image just presented and of retrieved106PALLER ET AL.。