有关锅炉英文文献
燃煤锅炉 外文翻译 外文文献 英文文献 中英翻译

Controlling the Furnace Process in Coal-Fired BoilersThe unstable trends that exist in the market of fuel supplied to thermal power plants and the situations in which the parameters of their operation need to be changed (or preserved), as well as the tendency toward the economical and environmental requirements placed on them becoming more stringent, are factors that make the problem of controlling the combustion and heat transfer processes in furnace devices very urgent. The solution to this problem has two aspects. The first involves development of a combustion technology and,accordingly, the design of a furnace device when new installations are designed. The second involves modernization of already existing equipment. In both cases,the technical solutions being adopted must be properly substantiated with the use of both experimental and calculation studies.The experience Central Boiler-Turbine Institute Research and Production Association (Ts KTI) and Zi O specialists gained from operation of boilers and experimental investigations they carried out on models allowed them to propose several new designs of multicell and maneuverable—in other words, controllable—furnace devices that had been put in operation at power stations for several years. Along with this, an approximate zero-one-dimensional, zone wise calculation model of the furnace process in boilers had been developed at the Tsk Ti, which allowed Tsk Ti specialists to carry out engineering calculations of the main parameters of this process and calculate studies of furnaces employing different technologies of firing and combustion modes .Naturally, furnace process adjustment methods like changing the air excess factor, stack gas recirculation fraction, and distribution of fuel and air among the tiers of burners, as well as other operations written in the boiler operational chart, are used during boiler operation.However, the effect they have on the process is limited in nature. On the other hand, control of the furnace process in a boiler implies the possibility of making substantial changes in the conditions under which the combustion and heat transfer proceed in order to considerably expand the range ofloads, minimize heat losses, reduce the extent to which the furnace is contaminated with slag, decrease the emissions of harmful substances, and shift to another fuel. Such a control can be obtained by making use of the following three main factors:(i) the flows of oxidizer and gases being set to move in the flame in a desired aerodynamic manner;(ii) the method used to supply fuel into the furnace and the place at which it is admitted thereto;(iii) the fineness to which the fuel is milled.The latter case implies that a flame-bed method is used along with the flame method for combusting fuel.The bed combustion method can be implemented in three design versions: mechanical grates with a dense bed, fluidized-bed furnaces, and spouted-bed furnaces.As will be shown below, the first factor can be made to work by setting up bulky vorticisms transferring large volumes of air and combustion products across and along the furnace device. If fuel is fired in a flame, the optimal method of feeding it to the furnace is to admit it to the zones near the centers of circulating vorticisms, a situation especially typical of highly intense furnace devices. The combustion process in these zones features a low air excess factor (α< 1) and a long local time for which the components dwell in them, factors that help make the combustion process more stable and reduce the emission of nitrogen oxides .Also important for the control of a furnace process when solid fuel is fired is the fineness to which it is milled; if we wish to minimize incomplete combustion, the degree to which fuel is milled should be harmonized with the location at which the fuel is admitted into the furnace and the method for supplying it there, for the occurrence of unburned carbon may be due not only to incomplete combustion of large-size fuel fractions, but also due to fine ones failing to ignite (especially when the content of volatiles Daff < 20%).Owing to the possibility of pictorially demonstrating the motion of flows, furnace aerodynamics is attracting a great deal of attention of researchers and designers who develop and improve furnace devices. At the same time, furnace aerodynamics lies at the heart of mixing (mass transfer), a process the quantitativeparameters of which can be estimated only indirectly or by special measurements. The quality with which components are mixed in the furnace chamber proper depends on the number, layout, and momenta of the jets flowing out from individual burners or nozzles, as well as on their interaction with the flow of flue gases, with one another, or with the wall.It was suggested that the gas-jet throw distance be used as a parameter determining the degree to which fuel is mixed with air in the gas burner channel. Such an approach to estimating how efficient the mixing is may to a certain degree be used in analyzing the furnace as a mixing apparatus. Obviously, the greater the jet length (and its momentum), the longer the time during which the velocity gradient it creates in the furnace will persist there, a parameter that determines how completely the flows are mixed in it. Note that the higher the degree to which a jet is turbulence at the outlet from a nozzle or burner, the shorter the distance which it covers, and, accordingly, the less completely the components are mixed in the furnace volume. Once through burners have advantages over swirl ones in this respect.It is was proposed that the extent to which once through jets are mixed as they penetrate with velocity w2 and density ρ2 into a transverse (drift) flow moving with velocity w1 and having density ρ1 be correlated with the relative jet throw distance in the following wayWhere ks is a proportionality factor that depends on the “pitch” between the jet axes (ks= 1.5–1.8).The results of an experimental investigation in which the mixing of gas with air in a burner and then in a furnace was studied using the incompleteness of mixing as a parameter are reported in 5.A round once through jet is intensively mixed with the surrounding medium in a furnace within its initial section, where the flow velocity at the jet axis is still equal to the velocity w2 at the nozzle orifice of radius r0.The velocity of the jet blown into the furnace drops very rapidly beyond the confines of the initial section, and the axis it has in the case of wall-mounted burners bends toward the outlet from the furnace.One may consider that there are three theoretical models for analyzing the mixing of jets with flow rate G2 that enter into a stream with flow rate G1. The firstmodel is for the case when jets flow into a “free” space (G1= 0),the second model is for the case when jets flow into a transverse (drift) current with flow rate G1 G2,and the third model is for the case when jets flow into a drift stream with flow rateG1<G2. The second model represents mixing in the channel of a gas burner, and the third model represents mixing in a furnace chamber. We assume that the mixing pattern we have in a furnace is closer to the first model than it is to the second one, since 0 <G1/G2< 1, and we will assume that the throw distance h of the jet being drifted is equal to the length S0 of the “free” jet’s initial section. The ejection ability of the jet being drifted then remains the same as that of the “free” jet, and the length of the initial section can be determined using the well-known empirical formula of G.N. Amphibrachic [6] :S0= 0.67r0/a, (2)where a is the jet structure factor and r0 is the nozzle radius.At a = 0.07, the length of the round jet’s initial section is equal to 10 r0 and the radius the jet has at the transition section (at the end of the initial section) is equal to 3.3 r0. The mass flow rate in the jet is doubled in this case. The corresponding minimum furnace cross-sectional area Ff for a round once through burner with the outlet cross-sectional area Fb will then be equal to and t he ratio Ff/Fb≈20. This value is close to the actual values found in furnaces equipped with once through burners. In furnaces equipped with swirl burners, a= 0.14 and Ff/Fb≈10. In both cases, the interval between the burners is equal to the jet diameter in the transition section d tr , which differs little from the value that has been established in practice and recommended in [7].The method traditionally used to control the furnace process in large boilers consists of equipping them with a large number of burners arranged in several tiers. Obviously, if the distance between the tiers is relatively small, operations on disconnecting or connecting them affect the entire process only slightly. A furnace design employing large flat-flame burners equipped with means for controlling the flame core position using the aerodynamic principle is a step forward. Additional possibilities for controlling the process in TPE-214 and TPE-215 boilers with a steam output of 670 t/h were obtained through the use of flat-flame burners arranged in two tiers with a large distance between the tiers; this made it possible not only to raise orlower the flame, but also to concentrate or disperse the release of heat in it [1]. A very tangible effect was obtained from installing multicell (operating on coal andopen-hearth, coke, and natural gases) flat-flame burners in the boilers of cogeneration stations at metallurgical plants in Ukraine and Russia.Unfortunately, we have to state that, even at present, those in charge of selecting the type, quantity, and layout of burners in a furnace sometimes adopt technical solutions that are far from being optimal. This problem should therefore be considered in more detail.If we increase the number of burners nb in a furnace while retaining their total cross-sectional area (ΣFb=idem) and the total flow rate of air through them, their equivalent diameters deq will become smaller, as will the jet momentums GB, resulting in a corresponding decrease in the jet throw distance Hb and the mass they eject. The space with high velocity gradients also becomes smaller, resulting in poorer mixing in the furnace as a whole. This factor becomes especially important when the emissions of Box and CO are suppressed right inside the furnace using staged combustio n (at αb < 1) under the conditions of a Fortinbras nonuniform distribution of fuel among the burners.In [1], a quantitative relationship was established between the parameters characterizing the quality with which once through jets mix with one another as they flow into a limited space with the geometrical parameter of concentration = with nb = idem and GB = idem. By decreasing this parameter we improve the mass transfer in the furnace; however, this entails an increase in the flow velocity and the expenditure of energy (pressure drop) in the burners with the same Fb. At the same time, we know from experience and calculations that good mixing in a furnace can be obtained without increasing the head loss if we resort to large long-range jets. This allows a much less stringent requirement to be placed on the degree of uniformity with which fuel must be distributed among the burners. Moreover, fuel may in this case be fed to the furnace location where it is required from process control considerations.For illustration purposes, we will estimate the effect the number of burners has on the mixing in a furnace at = = idem. schematically shows the plan views of two furnace chambers differing in the number of once through round nozzles (two andfour) placed in a tier (on one side of the furnace). The furnaces have the same total outlet cross-sectional areas of the nozzles (ΣF b) and the same jet velocities related to these areas (wb). The well-known swirl furnace of the TsKTI has a design close to the furnace arrangement under consideration. According to the data of [1], the air fraction βair that characterizes the mixing and enters through once through burners into the furnace volume beneath them can be estimated using the formula βair = 1 – (3) which has been verified in the range = 0.03–0.06 for a furnace chamber equipped with two frontal once through burners. Obviously, if we increase the number of burners by a factor of 2, their equivalent diameter, the length of the initial section of jets S0 and the area they “serve” will reduce by a factor of Then, for example, at = 0.05, the fraction βair will decrease from 0.75 to 0.65. Thus, Eq. (3) may be written in the following form for approximately assessing the effect of once through burners on the quality of mixing in a furnace:βair = 1 – 3.5f nb ' ,where is the number of burners (or air nozzles) on one wall when they are arranged in one tier both in onesided and opposite manners.The number of burners may be tentatively related to the furnace depth af (at the same = idem) using the expression (5)It should be noted that the axes of two large opposite air nozzles ( = 1)—an arrangement implemented in an inverted furnace—had to be inclined downward by more than 50° [8].One well-known example of a furnace device in which once through jets are used to create a large vortex covering a considerable part of its volume is a furnace with tangentially arranged burners. Such furnaces have received especially wide usein combination with pulverizing fans. However, burners with channels having a small equivalent diameter are frequently used for firing low-calorific brown coals with high content of moisture. As a result, the jets of air-dust mixture and secondary air that go out from their channels at different velocities(w2/w1 = 2–3) become turbulence and lose the ability to be thrown a long distance; as a consequence, the flame comes closer to the water walls and the latter are contaminated with slag. One method by which the tangential combustion scheme can be improved consists of organizing the so-called concentric admission of large jets of air-dust mixture and secondary air with the fueland air nozzles spaced apart from one another over the furnace perimeter, accompanied by intensifying the ventilation of mills [9, 10]. Despite the fact that the temperature level in the flame decreases, the combustion does not become less stable because the fuel mixes with air in a stepwise manner in a horizontal plane.V ortex furnace designs with large cortices the rotation axes of which are arranged transversely with respect to the main direction of gas flow have wide possibilities in terms of controlling the furnace process. In [1], four furnace schemes with a controllable flame are described, which employ the principle of large jets colliding with one another; three of these schemes have been implemented. A boiler with a steam capacity of 230 t/h has been retrofitted in accordance with one of these schemes (with an inverted furnace) . Tests of this boiler, during which air-dust mixture was fed at a velocity of 25–30 m/s from the boiler front using a high concentration dust system, showed that the temperature of gases at the outlet from the furnace had a fairly uniform distribution both along the furnace width and depth . A simple method of shifting the flame core over the furnace height was checked during the operation of this boiler, which consisted of changing the ratio of air flow rates through the front and rear nozzles;this allowed a shift to be made from running the furnace in adry-bottom mode to a slag-tap mode and vice Versace. A bottom-blast furnace scheme has received rather wide use in boilers equipped with different types of burners and mills. Boilers with steam capacities ranging from 50 to 1650 t/h with such an aerodynamic scheme of furnaces manufactured by ZiO and Bergomask have been installed at a few power stations in Russia and abroad . We have to point out that, so far as the efficiency of furnace process control is concerned, a combination of the following two aerodynamic schemes is of special interest: the inverted scheme and the bottom-blast one. The flow pattern and a calculation analysis of the furnace process in such a furnace during the combustion of lean coal are presented in [13].Below, two other techniques for controlling the furnace process are considered. Boilers with flame–stoker furnaces have gained acceptance in industrial power engineering, devices that can be regarded to certain degree as controllable ones owing to the presence of two zones in them . Very different kinds of fuel can be jointly combusted in these furnaces rather easily. An example of calculating such a furnacedevice is given in [2]. As for boilers of larger capacity, work on developing controllable two-zone furnaces is progressing slowly . The development of a furnace device using the so-called VIR technology (the transliterated abbreviation of the Russian introduction, innovation, and retrofitting) can be considered as holding promise in this respect. Those involved in bringing this technology to the state of industry standard encountered difficulties of an operational nature (the control of the process also presented certain difficulties). In our opinion, these difficulties are due to the fact that the distribution of fuel over fractions can be optimized to a limited extent and that the flow in the main furnace volume has a rather sluggish aerodynamic structure. It should also be noted that the device for firing the coarsest fractions of solid fuel in a spouting bed under the cold funnel is far from being technically perfect.Centrifugal dust concentrators have received acceptance for firing high-reactive coals in schemes employing pulverizing fans to optimize the distribution of fuel as to its flow rate and fractions. The design of one such device is schematically shown in [9]. Figure shows a distribution of fuel flow rates among four tiers of burners that is close to the optimum one. This distribution can be controlled if we furnish dust concentrators with a device with variable blades, a solution that has an adequate effect on the furnace process.燃煤锅炉的燃烧进程控制存在于火电厂的市场的燃料供应,某些操作参数需要改变(或保留)的情况下,以及经济和环境方面倾向的要求使他们变得更加严格的不稳定趋势是导致使控制燃烧与传热过程炉设备非常紧迫的主要因素。
外文翻译---电锅炉温度控制系统
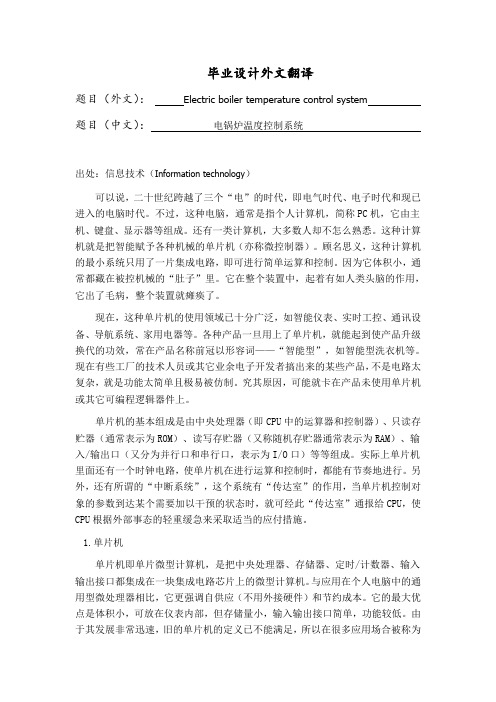
毕业设计外文翻译题目(外文):Electric boiler temperature control system题目(中文):电锅炉温度控制系统出处:信息技术(Information technology)可以说,二十世纪跨越了三个“电”的时代,即电气时代、电子时代和现已进入的电脑时代。
不过,这种电脑,通常是指个人计算机,简称PC机,它由主机、键盘、显示器等组成。
还有一类计算机,大多数人却不怎么熟悉。
这种计算机就是把智能赋予各种机械的单片机(亦称微控制器)。
顾名思义,这种计算机的最小系统只用了一片集成电路,即可进行简单运算和控制。
因为它体积小,通常都藏在被控机械的“肚子”里。
它在整个装置中,起着有如人类头脑的作用,它出了毛病,整个装置就瘫痪了。
现在,这种单片机的使用领域已十分广泛,如智能仪表、实时工控、通讯设备、导航系统、家用电器等。
各种产品一旦用上了单片机,就能起到使产品升级换代的功效,常在产品名称前冠以形容词——“智能型”,如智能型洗衣机等。
现在有些工厂的技术人员或其它业余电子开发者搞出来的某些产品,不是电路太复杂,就是功能太简单且极易被仿制。
究其原因,可能就卡在产品未使用单片机或其它可编程逻辑器件上。
单片机的基本组成是由中央处理器(即CPU中的运算器和控制器)、只读存贮器(通常表示为ROM)、读写存贮器(又称随机存贮器通常表示为RAM)、输入/输出口(又分为并行口和串行口,表示为I/O口)等等组成。
实际上单片机里面还有一个时钟电路,使单片机在进行运算和控制时,都能有节奏地进行。
另外,还有所谓的“中断系统”,这个系统有“传达室”的作用,当单片机控制对象的参数到达某个需要加以干预的状态时,就可经此“传达室”通报给CPU,使CPU根据外部事态的轻重缓急来采取适当的应付措施。
1.单片机单片机即单片微型计算机,是把中央处理器、存储器、定时/计数器、输入输出接口都集成在一块集成电路芯片上的微型计算机。
工业锅炉节能毕业论文中英文资料外文翻译文献

工业锅炉节能中英文资料外文翻译文献专业英文资料Boiler energy savingIndustrial boiler energy saving technology related to many, what is the most important increase industrial furnace thermal utilization ratio of pot namely, increasing the thermal efficiency of the industrial boiler. This section from burning, transportation line maintenance, new technology and new equipment and the application of the industrial boiler auxiliary equipment of energy saving, pot boiler water processing, etc and the industrial boiler room way of energy saving is discussed in this paper.1. The furnace of industrial boiler furnace arch arch is very important. The role is to make arch furnace chamber of the mixture of gases and radiation and hot gas organization flow, to make the fuel and ignition and combustion when. Sua and at present industrial boiler with the actual YongQiLiang rated load are often not with horse, the use of coal changes greatly, and often have large design coal poor vision, so in actual use, often to furnace necessary improvement in arch coal need to be comfortable.For transformation of the former furnace arch situation, the existing problem is: for the use of coal and coal than design poor miscellaneous, boiler flue gas temperature appear the chamber exports low (about 700℃), more than 200 ℃ design low. The new coal fire late, often appear fire bed broken fire, fire from about 0.6 ~ coal disc 1 cm, furnace combustion is not strong, ash high carbon content. According to the problems furnace arch structure, from improving the ignition of fuel conditions and raise the temperature of boiler furnace to reform.After improvement furnace arch, in actual shipped in from the observed, the transformation effect is good, people away from coal furnace fuel after disc 0.3 MRP on fire, fire bed combustion intense, flame full of degree good, strong rotation. Due to the lower arch before, after extended arch, the arch of the throat and mouth shape between into space from the original 2 cm or so down to 1 cm. To strengthen the disturbance of the air mixed, to form the airflow, strengthen the furnace combustion, improve the efficiency of the district and the whole arch before furnace temperature, make its reach to 1400℃ above, improved the ignition of fuel conditions. Coal in the ignition, of furnace temperature rise, make the carbon content and ash significantly less. The flue gas mixture and strengthen the hydrocyclone separation of flue gas carbon particles needed to fall in the fire bed and new fuel layer further burns out.2. The reasonable air supply and regulationIn the chain furnace, the furnace, the furnace of reciprocating vibration, according to the different characteristics of the combustion process, reasonable air supply, to promote the furnace combustion is very important. As in the chain furnace, along with fuel keep movement, which in turn happen on fire, burning, and burn the stage. Burning along the length direction is the stoker stages, zoning, so along the length direction along the air quantity is also different. The preheating zone along the head and tail burn stage, air requirements small; The burning along the middle stage, air requirements. According to this13characteristic, must use block supply air, to meet the needs of the burning. The current domestic production of the boiler although all are to consider this one characteristic, with the wind in subsection room, and equipped with air inlet adjustment. But according to the survey.3.The secondary airSecond wind to strengthen the air combustion is very effective. Second wind have the following function:(1) strengthen the furnace of air disturbance and mixed, make the furnace of oxygen and flammable gas mixture evenly, make chemical don't fully burning loss and the chamber excess air coefficient reducing. (2) secondary air in furnace flue gas vortex formed, on the one hand, extended the suspension fine coal grain in the chamber of a stroke, increase the fine particles suspended furnace in the residence time of, make it have a full time to burn, make not complete combustion heat loss; Another result of air separation of spiral effect, make coal dust grain and the grain re-blows rejection within, and reduce the small fly ash escape from the quantity, the mechanical incomplete combustion heat loss.英译汉14锅炉的节能工业锅炉的节能技术涉及多方面 , 最主要是提高工业锅炉的热能利用率 , 即提高工业锅炉的热效率。
锅炉运行论文
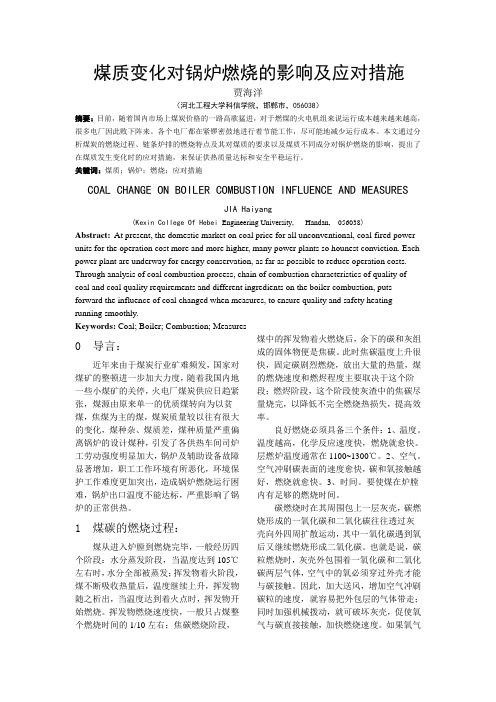
煤质变化对锅炉燃烧的影响及应对措施贾海洋(河北工程大学科信学院,邯郸市,056038)摘要:目前,随着国内市场上煤炭价格的一路高歌猛进,对于燃煤的火电机组来说运行成本越来越来越高,很多电厂因此败下阵来。
各个电厂都在紧锣密鼓地进行着节能工作,尽可能地减少运行成本。
本文通过分析煤炭的燃烧过程、链条炉排的燃烧特点及其对煤质的要求以及煤质不同成分对锅炉燃烧的影响,提出了在煤质发生变化时的应对措施,来保证供热质量达标和安全平稳运行。
关键词:煤质;锅炉;燃烧;应对措施COAL CHANGE ON BOILER COMBUSTION INFLUENCE AND MEASURESJIA Haiyang(Kexin College Of Hebei Engineering University, Handan, 056038)Abstract:At present, the domestic market on coal price for all unconventional, coal-fired power units for the operation cost more and more higher, many power plants so hounest conviction. Each power plant are underway for energy conservation, as far as possible to reduce operation costs. Through analysis of coal combustion process, chain of combustion characteristics of quality of coal and coal quality requirements and different ingredients on the boiler combustion, puts forward the influence of coal changed when measures, to ensure quality and safety heating running smoothly.Keywords: Coal; Boiler; Combustion; Measures0 导言:近年来由于煤炭行业矿难频发,国家对煤矿的整顿进一步加大力度,随着我国内地一些小煤矿的关停,火电厂煤炭供应日趋紧张,煤源由原来单一的优质煤转向为以贫煤,焦煤为主的煤,煤炭质量较以往有很大的变化,煤种杂、煤质差,煤种质量严重偏离锅炉的设计煤种,引发了各供热车间司炉工劳动强度明显加大,锅炉及辅助设备故障显著增加,职工工作环境有所恶化,环境保护工作难度更加突出,造成锅炉燃烧运行困难,锅炉出口温度不能达标,严重影响了锅炉的正常供热。
燃煤锅炉 外文翻译 外文文献 英文文献 中英翻译

燃煤锅炉外文翻译外文文献英文文献中英翻译外文出处: A. A. Shatil’, N. S. K. A. A. S., & V. Kudryavtsev, A. (2008). Controllingthe furnace process in coal-fired boilers. Thermal Engineering, 55, 1, 72-77.Controlling the Furnace Process in Coal-Fired BoilersThe unstable trends that exist in the market of fuel supplied to thermal power plants and the situations in which the parameters of their operation need to be changed (or preserved), as well as the tendency toward the economical and environmental requirements placed on them becoming more stringent, are factors that make the problem ofcontrolling the combustion and heat transfer processes in furnace devices very urgent. The solution to this problem has two aspects. The first involves development of a combustion technology and,accordingly, the design of a furnace device when new installations are designed. The second involves modernization of already existing equipment. In both cases,the technical solutions being adopted must be properly substantiated with the use of both experimental and calculationstudies.The experience Central Boiler-Turbine Institute Research and Production Association (TsKTI) and ZiO specialists gained from operation of boilers and experimental investigations they carried out on models allowed them to propose several new designs of multifuel andmaneuverable—in other words, controllable—furnace devices that had been put in operationat power stations for several years. Along with this, an approximate zero-one-dimensional, zonewise calculation model of the furnace process in boilers had been developed at the TsKTI, which allowed TsKTI specialists to carry out engineering calculations of the main parameters of this process and calculate studies of furnaces employing different technologies of firing and combustion modes .Naturally, furnace process adjustment methods like changing the air excess factor, stack gas recirculation fraction, and distribution offuel and air among the tiers of burners, as well as other operations written in the boiler operational chart, are used during boiler operation.However, the effect they have on the process is limited in nature. On the other hand, control of the furnace process in a boiler implies the possibility of making substantial changes in the conditions under which the combustion and heat transfer proceed in order to considerably expand the range of loads, minimize heat losses, reduce the extent to which the furnace is contaminated with slag, decrease the emissions of harmful substances, and shift to another fuel. Such a control can be obtained by making use of the following three main factors:(i) the flows of oxidizer and gases being set to move in the flamein a desired aerodynamic manner;1(ii) the method used to supply fuel into the furnace and the placeat which it is admitted thereto;(iii) the fineness to which the fuel is milled.The latter case implies that a flame-bed method is used along withthe flame method for combusting fuel.The bed combustion method can be implemented in three design versions: mechanical grates with a dense bed, fluidized-bed furnaces, and spouted-bed furnaces.As will be shown below, the first factor can be made to work bysetting up bulky vortices transferring large volumes of air and combustion products across and along the furnace device. If fuel isfired in a flame, the optimal method of feeding it to the furnace is to admit it to the zones near the centers of circulating vortices, a situation especially typical of highly intense furnace devices. The combustion process in the se zones features a low air excess factor (α< 1) and a long local time for which the components dwell in them, factors that help make the combustion process more stable and reduce theemission of nitrogen oxides .Also important for the control of a furnace process when solid fuelis fired is the fineness to which it is milled; if we wish to minimize incomplete combustion, the degree to which fuel is milled should be harmonized with the location at which the fuel is admitted into the furnace and the method for supplying it there, for the occurrence of unburned carbon may be due not only to incomplete combustion of large-size fuel fractions, but also due to fine ones failing to ignite (especially when the content of volatiles Vdaf < 20%).Owing to the possibility of pictorially demonstrating the motion of flows, furnace aerodynamics is attracting a great deal of attention of researchers and designers who develop and improve furnace devices. At the same time, furnace aerodynamics lies at the heart of mixing (mass transfer), a process the quantitative parameters of which can be estimated only indirectly or by special measurements. The quality with which components are mixed in the furnace chamber proper depends on the number, layout, and momenta of the jets flowing out from individual burners or nozzles, as well as on their interaction with the flow of flue gases, with one another, or with the wall.It was suggested that the gas-jet throw distance be used as a parameter determining the degree to which fuel is mixed with air in the gas burner channel. Such an approach to estimating how efficient the mixing is may to a certain degree be used in analyzing the furnace as a mixing apparatus. Obviously, the greater the jet length (and its momentum), the longer the time during which the velocity gradient it creates in the furnace will persist there, a parameter that determines how completely the flows are mixed in it. Note that the higher the degree to which a jet is turbulized at the outlet from a nozzle or burner, the shorter the distance which it covers, and, accordingly, the less completely the components are mixed in2the furnace volume. Once through burners have advantages over swirl ones in this respect.It is was proposed that the extent to which once through jets are mixed as they penetrate with velocity w2 and density ρ2 into a transverse (drift) flow moving with velocity w1 and having density ρ1 be correlated with the relative jet throw distance in the following way Where ks is a proportionality factor tha t depends on the ―pitch‖ between the jet axes (ks=1.5–1.8).The results of an experimental investigation inwhich the mixing of gas with air in a burner and then in a furnace was studied using the incompleteness of mixing as a parameter are reported in 5.A round once through jet is intensively mixed with the surrounding medium in a furnace within its initial section, where the flow velocity at the jet axis is still equal to the velocity w2 at the nozzle orifice of radius r0.The velocity of the jet blown into the furnace drops very rapidly beyond the confines of the initial section, and the axis it has in the case of wall-mounted burners bends toward the outlet from the furnace.One may consider that there are three theoretical models for analyzing the mixing of jets with flowrate G2 that enter into a stream with flowrate G1. The first model is for the case when jets flow into a ―free‖ space (G1= 0),the second model is for the case when jets flowinto a transverse (drift) current with flowrate G1G2,and the third model is for the case ,when jets flow into a drift stream with flowrate G1<G2. The second model represents mixing in the channel of a gas burner, and the third model represents mixing in a furnace chamber. We assume that the mixing pattern we have in a furnace is closer to the first model than it is to the second one, since 0 <G1/G2< 1, and we will assume that the throw distance h of the jet being drifted is equal to the length S0 of the―free‖ jet’s initial section. The ejection ability of the jet being drifted then remains the same as that of the ―free‖ jet, and the length of the initialsection can be determined using the well-known empirical formula of G.N. Abramovich [6] :S0= 0.67r0/a, (2)where a is the jet structure factor and r0 is the nozzle radius.At a = 0.07, the length of the round jet’s initial section is equal to 10 r0 and the radius the jet has at the transition section (at the end of the initial section) is equal to 3.3 r0. The mass flowrate in the jet is doubled in this case. The corresponding minimum furnace cross-sectional area Ff for a round once through burner with the outlet cross-sectional area Fb will then be equal to and the ratio Ff/Fb?20. This value is close to the actual values found in furnacesequipped with once through burners. In furnaces equipped with swirl burners, a= 0.14 and Ff/Fb?10. In both cases, the interval between theburners is equal to the jet diameter in the transition section d tr , which differs little from the value that has been established inpractice and recommended in [7].3The method traditionally used to control the furnace process inlarge boilers consists of equipping them with a large number of burners arranged in several tiers. Obviously, if the distance between the tiers is relatively small, operations on disconnecting or connecting themaffect the entire process only slightly. A furnace design employinglarge flat-flame burners equipped with means for controlling the flame core position using the aerodynamic principle is a step forward. Additional possibilities for controlling the process in TPE-214 and TPE-215 boilers with a steam output of 670 t/h were obtained through the use of flat-flame burners arranged in two tiers with a large distance between the tiers; this made it possible not only to raise or lower the flame, but also to concentrate or disperse the release of heat in it [1].A very tangible effect was obtained from installing multifuel (operating on coal and open-hearth, coke, and natural gases) flat-flame burners in the boilers of cogeneration stations at metallurgical plants in Ukraine and Russia.Unfortunately, we have to state that, even at present, those in charge of selecting the type, quantity, and layout of burners in a furnace sometimes adopt technical solutions that are far from being optimal. This problem should therefore be considered in more detail.If we increase the number of burners nb in a furnace while retaining their total cross-sectional area (ΣFb=idem) and the total flowrate ofair through them, their equivalent diameters deq will become smaller, as willthe jet momentums Gbwb, resulting in a corresponding decrease in the jet throw distance hb and the mass they eject. The space with high velocity gradients also becomes smaller, resulting in poorer mixing in the furnace as a whole. This factor becomes especially important whenthe emissions of NOx and CO are suppressed right inside the furnaceusing staged combustion (at αb < 1) under the conditionsof a fortiori nonuniform distribution of fuel among the burners.In [1], a quantitative relationship was established between the parameters characterizing the quality with which once through jets mix with one another as they flow into a limited space with the geometrical parameter of concentration = with nb = idem and Gb = idem. By decreasing this parameter we improve the mass transfer in the furnace; however,this entails an increase in the flow velocity and the expenditure of energy (pressure drop) in the burners with the same Fb. At the same time, we know from experience and calculations that good mixing in a furnace can be obtained without increasing the head loss if we resort to large long-range jets. This allows a much less stringent requirement to be placed on the degree of uniformity with which fuel must be distributed among the burners. Moreover, fuel may in this case be fed to the furnace location where it is required from process control considerations.For illustration purposes, we will estimate the effect the number of burners has on the mixing in a furnace at = = idem. schematically shows the plan views of two furnace chambers4differing in the number of once through round nozzles (two and four) placed in a tier (on one side of the furnace). The furnaces have the same total outlet cross-sectional areas of the nozzles (ΣFb) and the same jet velocities related to these areas (wb). The well-known swirl furnace of the TsKTI has a design close to the furnace arrangement under consideration. According to the data of [1], the air fraction βair that characterizes the mixing and entersthrough once through burners into the furnace volume beneath themcan be estimated using the formula βair = 1 – (3) which has been verified in the range = 0.03–0.06 for a furnacechamber equipped with two frontal once through burners. Obviously,if we increase the number of burners by a factor of 2, their equivalent diameter, the length of the initial section of jets S0 and the area they ―serve‖ will reduce by a factor of Then, for example, at = 0.05, the raction βair will decreas e from 0.75 to 0.65. Thus, Eq. (3) may be written in the following fform for approximately assessing the effect of once through burners on the quality of mixing in a furnace:βair = 1 – 3.5f nb ' ,where is the number of burners (or air nozzles) on one wall when they are arranged in one tier both in onesided and opposite manners.The number of burners may be tentatively related to the furnacedepth af (at the same = idem) using the expression (5)It should be noted that the axes of two large opposite air nozzles ( = 1)—an arrangementimplemented in an inverted furnace—had to be inclined downward by more than 50? [8].One well-known example of a furnace device in which once throughjets are used to create a large vortex covering a considerable part of its volume is a furnace with tangentially arranged burners. Such furnaces have received especially wide use in combination with pulverizing fans. However, burners with channels having a small equivalent diameter are frequently used for firing low-calorific brown coals with high content of moisture. As a result, the jets of air-dust mixture and secondary air that go out from their channels at different velocities(w2/w1 = 2–3) become turbulized and lose the ability to be thrown a long distance; as a consequence, the flame comes closer to the waterwalls and the latter are contaminated with slag. One method by which the tangential combustion scheme can be improved consists of organizing the so-called concentric admission of large jets of air-dust mixture and secondary air with the fuel and air nozzles spaced apart from one another over the furnace perimeter, accompanied by intensifying the ventilation of mills [9, 10]. Despite the fact that thetemperature level in the flame decreases, the combustion does not become less stable because the fuel mixes with air in a stepwise manner in a horizontal plane.Vortex furnace designs with large vortices the rotation axes ofwhich are arranged transversely with respect to the main direction of gas flow have wide possibilities in terms of5controlling the furnace process. In [1], four furnace schemes with a controllable flame are described, which employ the principle of large jets colliding with one another; three of these schemes have been implemented. A boiler with a steam capacity of 230 t/h has been retrofitted in accordance with one of these schemes (with an inverted furnace) . Tests of this boiler, during which air-dust mixture was fed at a velocity of 25–30 m/s from the boiler frontusing a highconcentration dust system, showed that the temperatureof gases at the outlet from the furnace had a fairly uniformdistribution both along the furnace width and depth . A simple method of shifting the flame core over the furnace height was checked during the operation of this boiler, which consisted of changing the ratio of air flowrates through the front and rear nozzles;this allowed a shift to be made from running the furnace in a dry-bottom mode to a slag-tap mode and vice versa. A bottom-blast furnace scheme has received rather wide use in boilers equipped with different types of burners and mills. Boilers with steam capacities ranging from 50 to 1650 t/h with such anaerodynamic scheme of furnaces manufactured by ZiO and Sibenergomash have been installed at a few power stations in Russia and abroad . We have to point out that, so far as the efficiency of furnace process control is concerned, a combination of the following two aerodynamic schemes is of special interest: the inverted scheme and the bottom-blast one. The flow pattern and a calculation analysis of the furnace process in such a furnace during the combustion of lean coal are presented in [13].Below, two other techniques for controlling the furnace process are considered. Boilers with flame–stoker furnaces have gained acceptancein industrial power engineering, devices that can be regarded to certain degree as controllable ones owing to the presence of two zones in them . Very different kinds of fuel can be jointly combusted in these furnaces rather easily. An example of calculating such a furnace device is given in [2]. As for boilers of larger capacity, work on developing controllable two-zone furnaces is progressing slowly . The development of a furnace device using the so-called VIR technology (thetransliterated abbreviation of the Russian introduction, innovation, and retrofitting) can be considered as holding promise in this respect. Those involved in bringing this technology to the state of industry standard encountered difficulties of an operational nature (the control of the process also presented certain difficulties). In our opinion, these difficulties are due to the fact that the distribution of fuel over fractions can be optimized to a limited extent and that the flow inthe main furnace volume has a rather sluggish aerodynamic structure. It should also be noted that the device for firing the coarsest fractions of solid fuel in a spouting bed under the cold funnel is far from being technically perfect.Centrifugal dust concentrators have received acceptance for firing high-reactive coals in schemes employing pulverizing fans to optimize the distribution of fuel as to its flowrate and6fractions. The design of one such device is schematically shown in [9]. Figure shows a distribution of fuel flowrates among four tiers of burners that is close to the optimum one. This distribution can be controlled if we furnish dust concentrators with a device with variable blades, a solution that has an adequate effect on the furnace process.7燃煤锅炉的燃烧进程控制存在于火电厂的市场的燃料供应,某些操作参数需要改变(或保留)的情况下,以及经济和环境方面倾向的要求使他们变得更加严格的不稳定趋势是导致使控制燃烧与传热过程炉设备非常紧迫的主要因素。
电厂锅炉英文文献
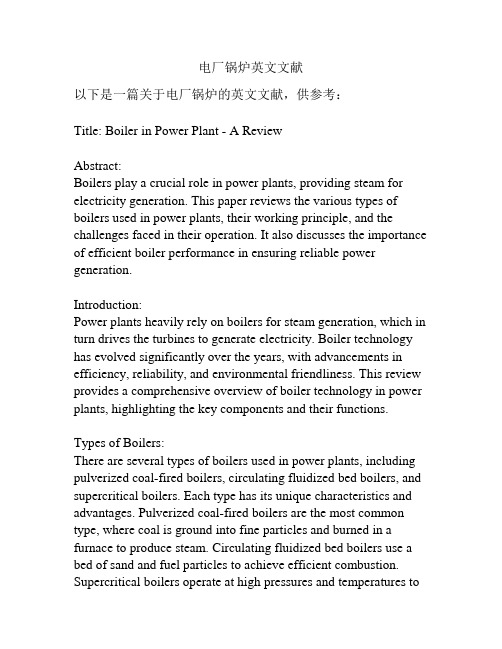
电厂锅炉英文文献以下是一篇关于电厂锅炉的英文文献,供参考:Title: Boiler in Power Plant - A ReviewAbstract:Boilers play a crucial role in power plants, providing steam for electricity generation. This paper reviews the various types of boilers used in power plants, their working principle, and the challenges faced in their operation. It also discusses the importance of efficient boiler performance in ensuring reliable power generation.Introduction:Power plants heavily rely on boilers for steam generation, which in turn drives the turbines to generate electricity. Boiler technology has evolved significantly over the years, with advancements in efficiency, reliability, and environmental friendliness. This review provides a comprehensive overview of boiler technology in power plants, highlighting the key components and their functions. Types of Boilers:There are several types of boilers used in power plants, including pulverized coal-fired boilers, circulating fluidized bed boilers, and supercritical boilers. Each type has its unique characteristics and advantages. Pulverized coal-fired boilers are the most common type, where coal is ground into fine particles and burned in a furnace to produce steam. Circulating fluidized bed boilers use a bed of sand and fuel particles to achieve efficient combustion. Supercritical boilers operate at high pressures and temperatures toimprove efficiency.Working Principle:Boilers operate on the principle of heat transfer from the combustion of fuel to the working fluid, typically water or steam. Fuel is burned in the furnace, generating heat that is transferred to the water/steam in the boiler tubes. The heat transfer process is facilitated by convection and radiation. The resulting steam is then used to drive the turbines for electricity generation.Challenges in Boiler Operation:Boiler operation faces several challenges, including maintaining high efficiency, controlling emissions, and ensuring safety. Efficiency is crucial as it directly impacts the overall power plant performance. Design and operational factors affect boiler efficiency, and continuous monitoring and optimization are required to maximize efficiency. Emission control is also a significant challenge, as power plants are subject to strict environmental regulations. Lastly, safety considerations are paramount to prevent accidents and ensure the well-being of personnel.Conclusion:Boilers are vital components in power plants, responsible for steam generation and subsequently electricity production. Understanding the different types of boilers, their working principles, and the challenges in their operation is essential for efficient and reliable power generation. Ongoing research and development in boiler technology aim to further improve efficiency, reduce emissions, and enhance safety in power plant operations.。
锅炉专业英语中英文对照表

锅炉专业英语中英文对照表火道锅炉Flame tube boiler疏水器、疏水阀steam trap饱和蒸汽saturated steam过热蒸汽superheated steam震动炉篦Oscillating bar grate链条炉蓖Chain grate, travelling grate省煤器economizer减速器speed reducer螺旋除渣机screw slag remover除尘器dust collectorcombustion air fan鼓风机exhausting fan上煤机coal conveyor尖端的、高科技的Hi-tech节能的energy-saving高效的high-efficiency低噪声的low noise耐用的、持久的durable蒸发evaporation额定的rated热效率thermal efficiency碳化物carbide碳化硅silicon carbidemonoxide一氧化碳carbon monoxide二氧化碳dioxide二氧化碳亚临界压力锅炉carbon dioxide subcritical pressure boiler燃煤锅炉coal-fired boiler启动锅炉 start-up boiler炉墙 furnace wall管束(排) tube bundle管屏tube platen下降管 downcomer上升管 riser省煤器管 economizer tube再热器管 reheater tube过热器管 superheated tube蛇形管 coil吊挂管 supporting tube水冷壁管 water wall tubesaturated steam pipe水冷壁 water wall鳍片管finned tube, fin tube, gilled tube 联箱 header锅炉本体 boiler proper锅炉机组 boiler unit炉膛 boiler framework燃烧器 furnace燃烧室 combustion chamber油枪 oil gun torch风门 damper管板 tube plate。
文献检索报告--热动11301

成绩:《文献检索与利用》实习报告论文题目:锅炉过热器姓名:陈磊班级:热动11301学号: 201124240141授课教师:于澄洁一、课题名称:中文题名:锅炉过热器英文题名:Boiler Superheater中文关键词:过热器英文关键词:Superheater二、课题概述:(约200~300字)锅炉过热器是把饱和蒸汽加热到额定过热温度的锅炉受热面部件。
回收锅炉烟气能量,使锅炉出来的蒸汽可以获得加热,变为干蒸汽,有利于提高锅炉热效率,也有利于蒸汽轮机避免水击。
过热器可根据布置位置和传热方式分为几类,一、对流式过热器,二、屏式过热器,三、辐射式过热器,四、炉顶过热器与包墙管过热器工业锅炉的过热器均为对流式过热器,现代大型电站锅炉的过热器则常由对流式,半辐射式和辐射式过热器组成。
三、《中国学术期刊全文数据库》()列举出部分检索结果(含摘要)。
1、锅炉过热器动态特性数理分析及其状态观测器的鲁棒性【作者中文名】韩忠旭【作者英文名】HAN Zhong-xu~(1,2) (1.China Electric Power Research Institute, Beijing 1000 85, China; 2. Beijing Stage Power Zhishen Control Technology Ltd. Beijing 100085, China) 【机构】中国电力科学研究院北京100085 北京国电智深控制技术有限公司;北京100085;【摘要】该文以过热器蒸汽温度受控对象为例,剖析了单相受热管焓-温通道的几种集总参数数学模型,它们共同的特性是特征方程的解均为实数根,从而具有过阻尼的性质。
在此基础上,进一步揭示应用高阶等容惯性环节作为锅炉过热器蒸汽温度受控对象的状态观测器这一方法内在的数学与物理基础,进而得出在设计锅炉过热器蒸汽温度受控对象的状态观测器时,不必受对象阶数的限制,通过调整观测器的参数,同样可以很好地完成重构状态并进行极点配置,起到改善受控系统动态特性的设计效果。
燃煤循环流化床锅炉外文翻译
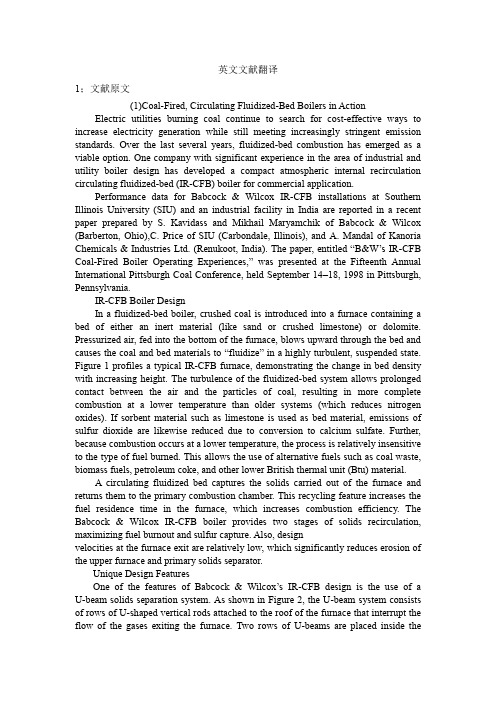
英文文献翻译1;文献原文(1)Coal-Fired, Circulating Fluidized-Bed Boilers in ActionElectric utilities burning coal continueto search for cost-effective ways toincrease electricity generation whilestill meeting increasingly stringent emissionstandards. Over the last several years,fluidized-bed combustion has emerged as aviable option. One company with significant experience in the area of industrial andutility boiler design has developed a compact atmospheric internal recirculation circulating fluidized-bed (IR-CFB) boiler forcommercial application.Performance data for Babcock & WilcoxIR-CFB installations at Southern IllinoisUniversity (SIU) and an industrial facility inIndia are reported in a recent paper preparedby S. Kavidass and Mikhail Maryamchik ofBabcock & Wilcox (Barberton, Ohio),C. Price of SIU (Carbondale, Illinois), andA. Mandal of Kanoria Chemicals & Industries Ltd. (Renukoot, India). The paper, entitled ―B&W’s IR-CFB Coal-Fired BoilerOpe rating Experiences,‖ was presented atthe Fifteenth Annual International Pittsburgh Coal Conference, held September 14–18, 1998 inPittsburgh, Pennsylvania.IR-CFB Boiler DesignIn a fluidized-bed boiler, crushed coal isintroduced into a furnace containing a bedof either an inert material (like sand orcrushed limestone) or dolomite. Pressurizedair, fed into the bottom of the furnace, blowsupward through the bed and causes the coaland bed materials to ―fluidize‖ in a highlyturbulent, suspended state. Figure 1 profilesa typical IR-CFB furnace, demonstrating thechange in bed density with increasingheight. The turbulence of the fluidized-bedsystem allows prolonged contact betweenthe air and the particles of coal, resulting inmore complete combustion at a lower temperature than older systems (which reducesnitrogen oxides). If sorbent material such aslimestone is used as bed material, emissionsof sulfur dioxide are likewise reduced due toconversion to calcium sulfate. Further, because combustion occurs at a lower temperature, the process is relatively insensitiveto the type of fuel burned. This allows theuse of alternative fuels such as coal waste,biomass fuels, petroleum coke, and otherlower British thermal unit (Btu) material.A circulating fluidized bed captures thesolids carried out of the furnace and returnsthem to the primary combustion chamber.This recycling feature increases the fuel residence time in the furnace, which increasescombustion efficiency. The Babcock &Wilcox IR-CFB boiler provides two stagesof solids recirculation, maximizing fuelburnout and sulfur capture. Also, designvelocities at the furnace exit are relativelylow, which significantly reduces erosion ofthe upper furnace and primary solids separator.Unique Design FeaturesOne of the features of Babcock & Wilcox’s IR-CFB design is the use of a U-beamsolids separation system. As shown in Figure 2, the U-beam system consists of rowsof U-shaped vertical rods attached to theroof of the furnace that interrupt the flow ofthe gases exiting the furnace. Two rows ofU-beams are placed inside the furnace itself,and four rows of U-beams are installed behind the furnace rear wallplane. The in-furnace U-beams capture about 75% of thesolids, which slide down the length of thebeams back into the combustion chamber.The remaining solid particles captured in theexternal U-beams are collected in a particlestorage hopper, which is periodically emptied back into the furnace forreburning.Theflue gas velocity across the U-beams isaround 8 meters/second (26.5 ft/sec) or less,producing a relatively low gas-side pressuredrop (less than 1 inch of water column) ascompared to conventional cyclone-typeseparators (6 to 10 inches of water column).The IR-CFB furnace is made of gas-tightmembrane enclosure water-cooled wallswith studded tubing spaced every fourinches. The lower furnace walls (up to aheight of 7.3 meters [24 ft]) are protectedwith an ultra high-strength, abrasion-resistant, low-cement refractory material lessthan 1 inch in thickness, which is placedover the studs protruding from the coolingtubes. A band of metal spray is typicallyapplied to further protect against erosion atthe point where the refractory material ends.The very thin application of refractory material means faster startup and less maintenance cost.Other beneficial characteristics of the IR-CFB boiler design include:* Use of in-furnace surfaces (division andwing walls) for furnace temperature control;* Gravity fuel feed and simplified secondary ash recycle system;* Absence of hot expansion joints, allowingsignificantly reduced maintenance;* Smaller footprint, which allows retrofitinside existing structural steel.Operating Experience at Two InstallationsThe IR-CFB design has been installed attwo locations—one at SIU in Carbondale, Illinois, and the second at the KanoriaChemicals & Industries Ltd. (Kanoria) sitein Renukoot, India. The SIU installation is a35-megawatt (MW) boiler that burns high-sulfur, low-ash Illinois coal, while the 81-MW Kanoria unit uses low-sulfur, high-ashcoal. The SIU boiler has a crushed limestonebed to combat the higher sulfur content ofthe fuel, while the Kanoria boiler uses a sandbed.SIU Unit dataThe SIU boiler is located close to the OldBen II coal mine in southern Illinois. Theplant was completed in 1996 and startedoperation in mid-1997. Performance testingwas completed in September 1997. Table 1shows the design and performance data forthe SIU boiler.Raw coal, delivered by truck, is movedby drag chain conveyor to a crusher. A24-hour capacity silo stores the pulverizedcoal. The coal is introduced into the furnaceby one gravimetric feeder through the sidewall. Two 60-MMBtu/hr gas-fired, over-bed burners and two 25-MMBtu/hr gas-fired, in-bed lances provide heat for startup.A multi-cyclone dust collector is used as asecondary solids separator (downstreamfrom the U-beams). The overall solids collection efficiency exceeds 90% and solidscollected in the cyclone are returned to thefurnace via an air fluidized conveyor. Abaghouse provides final particulate control.The bed material is periodically drainedfrom the furnace to control bed solids build-up and to remove any oversized material.The SIU unit has a single 8-inchdiameterdrain pipe to remove the bed, which iscooled with a screw ash cooler using recirculated plant water supply.Cold startup to 100% maximum continuous rating (MCR) can be achieved withinfive hours and the observed boiler dynamicload response is 5%–6% per minute. Aboiler turndown of 5:1 has been achievedwithout auxiliary fuel (a turndown ratio of3.5:1 to 4:1 is guaranteed). Further, all majorequipment has performed reliably whilemeeting or surpassing permitted emissions.A soot blower installed at the horizontalconvection pass floor has experienced plugging with ash and residual moisture. Whilethe boiler can operate successfully withoutthe soot blower, more investigation isneeded to overcome this operational glitch.Kanoria Unit DataThe Kanoria facility is located within thestate of Utter Pradesh, India, in close proximity to the Singaroli coal mine. The boilerwas constructed in 1996 and began commercial operation in February 1997. Performance testing continued until September1997. Design and performance data for theKanoria boiler are also shown in Table 1.In contrast to the Illinois coal, the Kanoriafuel is erosive, low in sulfur, and high in ash.Crushed coal is introduced via two volumetric drag chain feeders through the front wallof the furnace. Two 60-MMBtu/hr oil-firedover-bed burners provide heat for startup.Solids collected by the U-beams are reinjected by gravity into the furnace at fourlocations. The Kanoria unit uses an electro-static precipitator for final particulate control. Bed draining is accomplished throughtwo bed drain pipes and ash coolers; finematerial is returned to the furnace, whileoversize particles are diverted to the ashdisposal system.The observed boiler efficiency of 88.8%is higher than originally anticipated andcombustion efficiency has exceeded 99%,due to very low unburned carbon and lowflue gas outlet temperatures. However, theerosive nature of the fuel initially causedtubing leaks in the water-cooled furnacewall, which have been remedied by applyingadditional metal spray at the refractory interface and adjusting the interface angle.Also, furnace temperature exceeded designvalue on several occasions due to insufficient upper furnace inventory caused by failures of the first fields of the electrostaticprecipitator and the ash conveying system.Adjustments to the precipitator rectifier andthe ash silo backpressure have solved theseproblems.In summary, two examples of IR-CFBboilers are successfully operating at 100%MCR with varying fuel types. IR-CFB appears reliable and incorporates several verylow-maintenance features that reduce operating costs.(2)Why Build a Circulating Fluidized Bed Boilerto Generate Steam and Electric PowerAbstractIn Asia, demand for electric power continues to rise steeplydue to population growth, economic development, and progres-sive substitution of alternate technologywith clean forms ofenergy generation. Atmospheric circulating fluidized bed (CFB)echnology has emerged as an environmentally acceptable technology for burning a wide range of solid fuels to generate steamand electricity power. CFB, although less than 20 years old, is amature technology with more than 400 CFB boilers in operation worldwide, ranging from 5 MW e to 250 MW e.Electric utilities and Independent Power Producers must nowselect a technology that will utilize a wide range of low-costsolid fuels, reduce emissions, reduce life cycle costs, and provide reliable steam generation for electric power generation.Therefore, CFB is often the preferred technology. Even thoughpulverized coal (PC) fired boilers continue to play a major roleworldwide, they have inherent issues such as fuel inflexibility,environmental concerns and higher maintenance costs.This paper discusses the benefits of CFB boilers for utilityand industrial applications. Specific emphasis is given to B&W’snternal Recirculation CFB (IR-CFB) technology, CFB technology comparisons, PC vs. CFB technology, emissions benefits,and economics including maintenance cost and boiler reliabilty. IntroductionBabcock & Wilcox (B&W) is a leading global supplier ofindustrial/utility boilers and has supplied more than 700 unitstotaling more than 270,000 MW e. Many of B&W’s CFB boilerdesign features have been adapted from vast experience designing and building boilers of all types and sizes for industrial andelectric utility applications. B&W’s design is an inherently compact, distinctive internal recirculation fluidized bed (IR-CFB)boiler featuring U-Beam solids separators. The furnace and convection pass of the IR-CFB boiler are within a single, gas–tightmembrane enclosure as commonly found in Pulverized Coal(PC) fired boilers. This CFB technology has been successfullyintroduced in the global market.To date, B&W, including B&W joint ventures and licenseecompanies, has sold 16 CFB boilers worldwide, shown in Table 1.B&W offers IR-CFB boilers up to 175 MWe, both reheat andnon-reheat, with full commercial guarantees and warranties. TheIR-CFB boiler is simple in configuration and compact, requiresa smaller boiler foot print, has minimal refractory, requires lowmaintenance, features quick startup, and provides high avail-ability.The modern way of burning solid fuels requires fuel flex-ibility and reliable technology, plus good combustion efficiencywith low emissions. CFB technology is well suited for a widerange of sold fuels. CFB technology is proven, mature and competitive.What is CFB technology?CFB technology utilizes the fluidized bed principle in whichcrushed (6 –12 mm x 0 size) fuel and limestone are injectedinto the furnace or combustor. The particles are suspended in astream of upwardly flowing air (60-70% of the total air) whichenters the bottom of the furnace through air distribution nozzles.The balance of combustion air is admitted above the bottom ofthe furnace as secondary air. While combustion takes place at840-900 C, the fine particles (<450 microns) are elutriated outof the furnace with flue gas velocity of 4-6 m/s. The particlesare then collected by the solidsseparators and circulated back into the furnace. This combustion process is called circulatingfluidized bed (CFB). The particles’ circulation provides efficient heat transfer to the furnace walls and longer residence timefor carbon and limestone utilization. Similar to PC firing, thecontrolling parameters in the CFB combustion process are temperature, residence time and turbulence.Designers and power plant operators have vast experience in PC-fired boiler design and operations. Adapting and under-standing CFB technology by those familiar with the PC environment requires time. CFB technology brings the capability ofdesigns for a wide range of fuels from low quality to high quality fuels, lower emissions, elimination of high maintenance pulverizers, low auxiliary fuel support and reduced life cycle costs.A PC vs. IR-CFB comparison is given in Table 2.The combustion temperature of a CFB (840-900 C) is muchlower than PC (1350-1500 C) which results in lower Nox for-mation and the ability to capture SO2with limestone injectionin the furnace. Even though the combustion temperature of CFBis low, the fuel residence time is higher than PC, which resultsin good combustion efficiencies comparable to PC. The PC pulverizers, which grind the coal to 70% less than 75 microns, require significant maintenance expenses. These costs are virtually eliminated in CFB because the coal is crushed to 12 - 6 mmx 0 size. Even though CFB boiler equipment is designed forrelatively lower flue gas velocities, the heat transfer coefficientof the CFB furnace is nearly double that of PC which makes thefurnace compact. In an IR-CFB, auxiliary fuel support is neededfor cold startup and operation below 25% versus 40-60% MCRwith PC. One of the most important aspects is that CFB boilers release very low levels of SO2 and NO x pollutants compared to PC, as shown in Table 2. PC units need a scrubber system, whichrequires additional maintenance.CFB is a fuel-driven and flexible technologyCFB can be the technology of choice for several reasons.The CFB can handle a wide range of fuels such as coal, wastecoal, anthracite, lignite, petroleum coke and agricultural waste,with low heating value (>1500 kcal/kg), high moisture content(< 55%), and high ash content (< 60%). The fuel flexibility provides use of opportunity fuels where uncertainty of fuel supplyexists and economics are an issue. If a CFB boiler is designedfor coal, the same boiler can be used to burn lignite or petroleum coke or anthracite. The material handling and feeding system should be properly designed to meet these fuel variations.Such fuel flexibility is not available in the competing conventional PC-fired boiler technologies. This is one of the importantfeatures of CFB that the customer needs to analyze carefullybefore selecting a technology.Environmental benefits of CFB technologyThe CFB combustion process facilitates steam generationfiring a wide range of fuels while meeting the required emissions such as sulfur dioxide (SO2 ) and nitrogen oxides (NO x)even more effectively than World Bank guidelines, as shown inTable 3.The major environmental benefit of selecting CFB technology is the removal of SO2(90-95%) and NO x(emission is lessthan 100 ppm) in the combustion process without adding postcombustion cleaning equipment such as wet or dry flue gasdesulfurization (FGD) systems and selective catalytic reduction(SCR) systems. When the limestone is injected into the furnace,the following reactions occur.* Oxidation of sulfurS+O2 --> SO2* Limestone is calcined to form calcium oxideCaCO3--> CaO + CO2–425 kcal/kg (of CaCO3 )* Sulfur dioxide gas reacts with solid CaOSO2+ 1/2 O2 + CaO --> CaSO4 (Solid) +3740 kcal/kg (of S) The resulting calcium-sulfate-based ashes are chemicallystable and are easily disposed. This ash can be used as raw material for cement manufacturing, soil stabilization, concreteblocks, road base, structural fills, etc. Limestone injection isrequired for fuels with sulfur greater than >0.5%. Lime (CaO)and unburned carbon content must be considered in re-use applications, depending on the fuel being fired.NO x present in flue gas generally comes from two sources:the oxidation of nitrogen compounds in the fuel (fuel NO x) andreaction between the nitrogen and oxygen in the combustionair (thermal NO x). With low temperature and staged combustion,the oxidation of fuel nitrogen is suppressed resulting in verylow NO x emissions. NO x emissions are <100 ppm with CFB.CO and hydrocarbon emissions in the CFB boiler are wellcontrolled. In recent years, financial institutions have pushedthe power project developers to meet the World Bank emissionsrequirements. Therefore obtaining the project permit is less difficult with CFB technology.Design features of B&W IR-CFB Boiler technologyB&W IR-CFB technology is very comparable to PC-firedboilers in arrangement. The IR-CFB boiler design consists ofthe following major systems, shown in Fig. 1. The main CFBboiler components are:* Boiler furnace* Furnace bottom air distributor and nozzles* Primary solids separators and recirculation system* Secondary solids separators and recirculation system* Pendant superheater / reheater* Economizer and horizontal tubular air heater* Air assisted gravity fuel /limestone feed systemBoiler FurnaceThe furnace cross section is selected based on flue gas superficial velocity. B&W typically uses furnace depths of 3.7 m,4.6 m and 5.4 m, depending on the unit size. The furnace enclosure is made of gas-tight membrane water-cooled walls having63.5 mm or 76 mm tube diameters on 102 mm centers. The furnace primary zone is reduced in plan area cross section to provide good mixing and promote solids entrainment at low load.The auxiliary startup burners, fuel feed points and secondaryash re-injection (multicyclone/MDC) points are located in thisregion.A thin layer of refractory is applied on all lower furnace walls,including the lower portion of the division walls and wing wallnose to protect against corrosion and erosion. An ultra highstrength abrasion-resistant low cement alumina refractory 16-25 mm thick is applied over a dense pin studded pattern. B&Whas patented aRDZ TM reduced diameter zone feature that elimihas nates erosion concern at the furnace interface. The furnace temperature is precisely controlled by maintaining proper inventory and thus the combustion efficiency and the limestone utili-zation are maximized.Air Distrbutors and NozzlesThe furnace bottom air plenum or wind box is made of water-cooled panels or casing depending on startup air temperature. Bubble caps are fitted on the water-cooled distributor floorpanels as shown in Fig. 2. The bubble caps are designed to distribute air uniformly, prevent the back sifting of solids at lowload operation, and create good turbulence for fuel /sorbentmixing in the primary zone. The bubble caps are spaced 102mm x 117 mm with 60-70% of total combustion air admittedthrough the bottom. The balance 30-40% of total air is admittedthrough overfire nozzles (high velocity) in the front and rearfurnace walls.Primary Solids SeparatorsThe solids separation system is a key element of any CFBboiler design. The B&W separation system is designed for thelife of the unit without replacement, influencing life cycle costs.The B&W IR-CFB has a two stage primary solids separator asshown in Fig. 3, comprised of in-furnace U-Beam separatorsand external U-Beam separators. The in-furnace U-Beams (tworows) are able to collect nearly 75% of the solids. The remaining solids are collected by the four rows of external U-Beamsand are discharged from the hopper directly into the furnace throughthe transfer hopper located beneath the external U-Beams (See Fig.4). The flue gas velocity across the U-Beams is approximately 8-10 m/s, limiting the gas-side pressure drop to 0.25 kPa as compared with a typical cyclone separator’s pressure drop of 1.5 to 2.0kPa. A commercially available, high-grade stainless steel materialis used for the U-Beam separators.Secondary Solids SeparatorThe multicyclone dust collector (MDC) is located in the convective pass either upstream or downstream of the economizer.The MDC typically has a top inlet and top outlet as shown inFig. 5. The MDC tube diameter is normally 229 mm arrangedover the second pass entire cross section. The MDC providesoutstanding retainment of fine particles up to 50 microns. TheMDC collection tubes and spin vanes have high hardness (550BHN), designed for longer life and easy replacement duringplanned outages.The small quantities of fines which escape from the externalU-Beams are collected by the MDC. The collected fines arestored in the MDC hopper. Variable speed rotary feeders or inclinedscrews are used to control the ash recycle flow rate fromthe hopper. Precise furnace temperature control is achieved byadjusting the speed of the rotary feeders or inclined screws, taking the temperature signal from the furnace.The superheater may consist of vertical pendant type primary and secondary banks, located in the convection pass, aswell as surface in the furnace in the form of superheater wingwalls. An attemperator is used to control the final steam temperature over the design load range. The flue gas velocities arerelatively low and selected byconsidering the dust loading andash erosivity of the fuel. When required, the reheater is locatedin the convection pass, and steam bypass is recommended tocontrol the final reheater temperature.Economizer and Horizontal Tubular air heaterThe economizer is designed with tubes running front to backin an in-line arrangement. Flue gas velocities used consider thedust loading and ash erosivity of the fuel. If the MDC is locatedupstream of the economizer, higher velocities are used and boththe economizer and the air heater are located in an in-line arrangement to minimize ash fouling. The air heater is locatedafter the MDC and the economizer. The flue gas is outside thetubes and air is passed through the tubes. A hopper is providedat the bottom of the air heater and the ash collected in the hopper is purged to the ash disposal system. The tube material andflue gas velocities are selected by considering the dust loadingand the ash erosivity of the fuel. A steam coil air heater (SCAH)is used to protect the cold end of the air heater if required.Air-Assisted Gravity Fuel/Limestone Feed SystemFuel handling and feeding is one of the major challenges inCFB boiler operation, especially with waste fuels because ofhigh fines and moisture content. The crushed fuel (6-12 mm x0) is stored in the silo, usually located in front of the boiler asshown in Fig. 6. Fuel is fed to the boiler via down spout fromsilo discharge to feeder and a series of feeders and gravity feedchutes. The fuel chute will have at least a 65 degree angle fromhorizontal. Primary air is used to sweep the fuel into the furnace and as seal air to the feeders. The number of feed points isset to achieve even fuel distribution in the furnace.The limestone handling and feeding system is relativelysimple compared to the fuel feed system. Limestone is fed either pneumatically or mechanically into the CFB boiler. Thepneumatic system feeds the limestone directly into the furnacethrough furnace openings in the front and rear walls. In themechanical system, the limestone is fed into the discharge endof the fuel feeders via rotary feeders. The limestone falls bygravity down the fuel feed chute with the fuel into the furnace,and is a function of fuel velocity and required emissions.CFB Technology ComparisonVirtually all major boiler manufacturers are involved inCFB technology. Two distinct types of solids separation systems are used. One type is cyclone–based, which provides singlestage solids collection systems, and the second type is impactseparator-based, which provides a two-stage solids separationsystem. A comparison of B&W IR-CFB technology features withthose of other major commercial CFB technologies is given inTable 4.Advantages of the B&W IR-CFB Boiler technology*Boiler is compact with primary U-Beam separators andprovides internal solids recycle.* Boiler has a smaller foot print (up to 20 to 30% less building volume compared to a hot cyclone-based CFB boiler)* Boiler design is especially suitable for retrofitting of olderPC-fired boilers within the existing support steel.* Two-stage solids separation efficiency (>99.7%) provideshigher carbon efficiencies and better limestone utilization through higher solids residence time.* Wide turndown ratio (4:1) without auxiliary fuel is possible due to the selection of furnace velocity and control-lable solids recycle.* Less refractory in the boiler allows for quicker startupand lower maintenance costs.Economics of CFB TechnologyCFB technology can burn a wide range of low cost solidfuels and competes well with oil/gas fired plants. The decision-makers often ask, ―What are capital and operating costs andbenefits of a CFB boiler?‖ The experience in Europe and NorthAmerica suggests that for a sulfur fuel (>0.5%S) and less than150 MW, a CFB boiler has 8-15 percent lower capital costs aswell as 5-10% lower operating costs than a PC-fired boiler because of the FGD system. In general, CFB-based power plantsprovide low emissions control costs and low O&M costs, whichlead to lower life cycle costs. In the end, owner profit marginincreases and payback period improves as shown in Table 5.Costs not included in Table 5 are items such as land, projectdevelopment, permitting, escalation, taxes and owner’s costs,since these costs are common for both PC and CFB-based powerplants.Reliability of CFB TechnologyCFB boiler design is simple and proven, compared to othertechnologies. Experience indicates that operating and maintenance costs are relatively lower than PC-fired boilers becauseof the ability to burn lower rank fuels, thus reducing fuel costescalation uncertainty. Since maintenance areas are very minimal in the CFB boiler, the availability of the boiler is relativelyhigher. The CFB design allows emissions reduction without significant capital cost, since SO2 and NO x removal are inherentwithin the combustion process.ConculusionEven though a number of competing technologies areavailable in the market for steam and electric power generation, CFB is an excellent choice due to its fuel flexibility, widerturndown without support oil/gas, superior environmental performance, lower operating and maintenance costs, and safe, reliable and simple boiler operation. The B&W IR-CFB boilerdesign offers compact, superior performance due to two-stagesolids separation, and is cost effective for multiple fuel firingin both PC retrofit and greenfield applications.2;译文(1)燃煤循环流化床锅炉运行情况燃煤发电行业继续搜寻成本效益的方式,以增加发电量,同时达到日益严格的排放标准. 过去几年,流化床燃烧已成为一种可行的选择. 一个公司有重大领域的经验,工业及电站锅炉设计开发了紧凑型大气内部循环流化床(红外CFB )锅炉投入商业应用.Babcock & Wilcox的红外流化床装置, 最近在南伊利诺伊大学和印度的一个工业设施中,在一份由S.kavidass和米哈伊尔Maryamchik的Babcock &Wilcox (巴伯顿,俄亥俄州) , C.SIU(代尔,伊利诺州) ,和A的[27]卡诺里亚&化学工业有限公司( renukoot ,印度) 被报道 . 这份文件题为"巴威的红外循环流化床燃煤锅炉的操作经验" ,并提交于15年度国际匹兹堡煤炭会议于9月14-18 , 1998年在匹兹堡,宾夕法尼亚州.红外型循环流化床锅炉设计在循环流化床锅炉,煤粉被引入炉膛内的床上,其中包含或惰性物质(如砂或粉碎石灰石)或白云石。
锅炉设计外文介绍
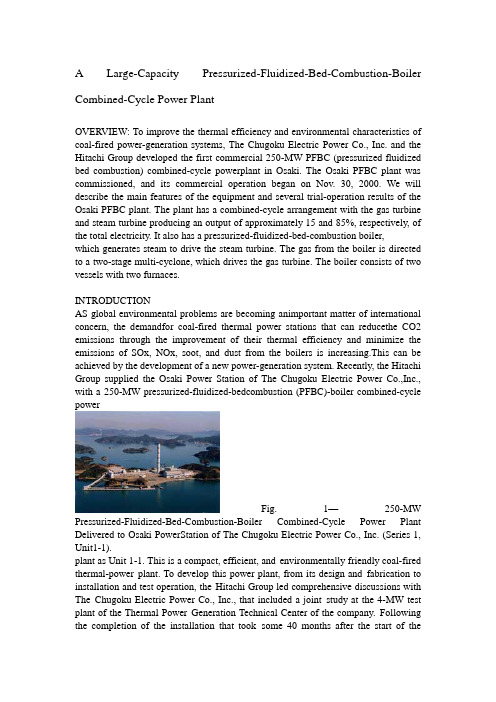
A Large-Capacity Pressurized-Fluidized-Bed-Combustion-Boiler Combined-Cycle Power PlantOVERVIEW: To improve the thermal efficiency and environmental characteristics of coal-fired power-generation systems, The Chugoku Electric Power Co., Inc. and the Hitachi Group developed the first commercial 250-MW PFBC (pressurized fluidized bed combustion) combined-cycle powerplant in Osaki. The Osaki PFBC plant was commissioned, and its commercial operation began on Nov. 30, 2000. We will describe the main features of the equipment and several trial-operation results of the Osaki PFBC plant. The plant has a combined-cycle arrangement with the gas turbine and steam turbine producing an output of approximately 15 and 85%, respectively, of the total electricity. It also has a pressurized-fluidized-bed-combustion boiler,which generates steam to drive the steam turbine. The gas from the boiler is directed to a two-stage multi-cyclone, which drives the gas turbine. The boiler consists of two vessels with two furnaces.INTRODUCTIONAS global environmental problems are becoming animportant matter of international concern, the demandfor coal-fired thermal power stations that can reducethe CO2 emissions through the improvement of their thermal efficiency and minimize the emissions of SOx, NOx, soot, and dust from the boilers is increasing.This can be achieved by the development of a new power-generation system. Recently, the Hitachi Group supplied the Osaki Power Station of The Chugoku Electric Power Co.,Inc., with a 250-MW pressurized-fluidized-bedcombustion (PFBC)-boiler combined-cycle powerFig. 1—250-MW Pressurized-Fluidized-Bed-Combustion-Boiler Combined-Cycle Power Plant Delivered to Osaki PowerStation of The Chugoku Electric Power Co., Inc. (Series 1, Unit1-1).plant as Unit 1-1. This is a compact, efficient, and environmentally friendly coal-fired thermal-power plant. To develop this power plant, from its design and fabrication to installation and test operation, the Hitachi Group led comprehensive discussions with The Chugoku Electric Power Co., Inc., that included a joint study at the 4-MW test plant of the Thermal Power Generation Technical Center of the company. Following the completion of the installation that took some 40 months after the start of theconstruction work in November 1995, the overall test operation began in March, 1999. The plant was put into commercial operation in November, 2000.This article describes the 250-MW PFBC powerplant project and the results of the test operation of the plant 1,2).Fig. 1 sh ows a bird’s-eye view of The Chugoku Electric’s Osaki Power Station that houses the main equipment fabricated by the Hitachi Group. Figs. 2 and 3 show the main equipment.The boiler is a twin-furnace type. The steam turbine and gas turbine are laid out in a T shape.250-MW PFBC PLANT EQUIPMENT General System Configuration The general system configuration of the plant is shown in Fig. 4, and the principal specifications of the plant are listed in Table 1. Unit 1-1 that was delivered to The Chugoku Electric’s Osaki Power Station is a combined-cyclepower-generation system, in which the steam turbine is driven by steam generated by a PFBC boiler housed in a pressure vessel and the gas turbine is driven by gas from which the dust is removed by two series of 12 two-stage cyclones (a multi-cyclone housed in an exclusive pressure vessel). By using limestone as the boiler-bed material, we could desulfurize the furnace gas and significantly reduce the SOx emissions. The boiler is a twin-furnace, twin-tower type, and the gas turbine is a single-shaft type. The fuel coal is fed intothe boiler in the form of coal-water paste (CWP), a mixture of coal, limestone that serves as the desulfurizing agent and bed material, and water.The Main Equipment BoilerThe fuel is burned under an in-furnace pressure of approximately 1 MPa, whereby the hearth load is increased and the required furnace cross-section area is reduced (i.e., the furnace is compact). Appropriate steps were taken to ensure uniform flow, stability, temperature uniformity, and so on, of the fluidized bed. They include the use of inclined front- and rear-end walls, a uniform arrangement of the heat-exchanger tubes in the furnace, and the installation of many airdistribution nozzles in the hearth at equal intervals3). In the fuel-supply system, a mixture of fuel coal, limestone, and water (CWP), prepared outside the pressure vessel, is fed into the fluidized-bedcombustion furnace inside the pressure vessel. This system reduced the number of through pipes in the pressure vessel and improved the safety of the furnace. To enable good controllability of the boiler operation and easy transportation and installation of the boiler (the project site is located on an island in the Inland Sea of Seto), we developed a twin-furnace, twin-tower boiler (“W.B.”). This boiler was shipped in the formof modules from the Kure Works of Babcock-Hitachi K.K.Fig. 3—Steam Turbine (Bottom) and Gas Turbine (Top) of the Power Station.The fluidized-bed temperature is 865°C. The bedheight varies from 2.5 to 4.0 m to enable changing theeffective area of the heat-exchanger tubes laid out inthe bed and adjusting the amount of steam generatedby the boiler. The flue gas from the boiler is first cleaned from soot and dust by two-stage cyclones until the soot and dust concentration drops below 1,000 mg/ m3N and is then fed to the gas turbine.Sulfur oxides (SOx) in the furnace gas aredesulfurized by the limestone in the furnace and thelimestone fed into the furnace together with coal. The formation of nitrogen oxides (NOx) is prevented by low-temperature combustion and the emissions of NOx are reduced by the use of flue-gas denitrification equipment (catalytic and non-catalytic denitrification). Thus, the boiler is environmentally friendly.Gas turbine and steam turbineThe gas turbine is similar to the one installed at The Chugoku Electric’s Yanai Power Station but was modified for PFBC. To ensure that the turbine blades can withstand the coal-ash-bearing hot gas (approximately 840°C) from the boiler, the thickness of both the rotating blades and stationary blades was increased and the blade coating characteristics were improved. A combination of an exclusive motor and exclusive combustors is used to start the gas turbine. The amount of air fed into the boiler is controlled by a compressor inlet pressure-reducing valve installed in the gas-turbine-compressor air-inlet duct and a guide vane installed at the compressor air inlet.The steam turbine uses a heat cycle whereby the gas-turbine waste heat is recovered by the steamturbine condensate and feed-water system. In addition, to improve the plant thermal efficiency, a TCDF-33.5 steam turbine that can be operated under large loads wasadopted.Fig. 4—General System Configuration of the 250-MW PFBC Power Plant. Fuel, compressed air, and water are fed into the twin-furnace, twin-tower boiler. As the fuel burns in the boiler, steam and high-pressure gas are generated.TABLE 1. Principal Specifications of the 250-MW PFBC Power Plant This plant uses a “two stage cyclone + bag filter” system, not a conventional ceramic-filter system, to remove soot and dust from the boiler flue gas.Fig. 5—Central Control Room. The central control room contains an automation panel, a large screen, and auxiliary panels for independent operation(1) The control system is composed of a central monitor panel, computers, and controls. The central monitor panel consists of an automation panel equipped with five CRT displays, a large screen, and auxiliary panels for independent operation. Centralized operations, including the operation of electrical equipment, are all performed by means of the CRT displays. To enable handling huge volumes of monitor information, we used a high-function, highperformanceCRT processor (see Fig. 5).(2)In automating the control operations, the control functions were distributed between the control computers and control devices for optimum results All plant operations, from the start of the seawater system to the operation of the plant under a full load to the shut-down of the plant, were made sequential on a system-by-system basis to implement a fullyautomated plant based on control computers. For routine operations, we adopted a remote, sequential control to minimize labor costs in plant operation.(3) For the main control equipment, such as the boiler control, turbine control, and sequence control, the HIACS-7000 system was used to configure horizontally-distributed control systems. The integration of controls by using a large-capacity, highperformance controller and the use of a high-speed data-transmission network (100 Mbit/s) enabled implementing a CRT-based operation of the entire plant and establishing a good human interface.(4) Instead of a conventional wired interlock of auxiliary devices, a programmable control module (PCM) and a remote process-input-and-output (PI/O) device were used. This significantly reduced the number of auxiliary relay panels and converter panels and enabled flexible response to field modifications. Because the remote PI/O devices were installed in thefield, the cable work could be reduced dramatically.TEST-OPERATION RESULTSPlant Performance To evaluate the plant performance during the hours of a season in which the atmospheric temperature at the site of the plant is almost the same as the atmospheric temperature for which the plant was designed, in May 2000, the plant was subjected to test operation under a rated output of 250 MW. We found that every piece of equipment of the plant met its planned output and efficiency. All the functions of the PFBC boiler worked as planned, including the infurnace desulfurization, non-catalytic denitrification (injection of an ammonia gas into the hot gas pipe at the furnace outlet), dust removal by two series of twostage multicyclones, etc. The planned figures for the emissions of NOx, SOx, and soot and dust were met completely (see Table 2).Plant Starting/Stopping The characteristics of the process of starting the plant in a cold mode, from the start of the gas turbine to the attainment of a rated load, are shown in Fig. 6.TABLE 2. Plant Performance (Planned Figures vs. Measured Results) Both the environmental-performance and thermal-efficiency results met the planned figurescompletely.Fig. 6— Measured Cold-Start Characteristics (Nov. 9-10, 2000). After the gas turbine is started, fuel is fed into the boiler to generate steam, which drives the steamturbine.Fig. 7— Plant Behavior at Load Change (May 11, 2000).One of the characteristics of the process of starting a PFBC plant is that once the plant equipment is synchronized with the electric power system, the gas turbine generator is operated as a synchronous motor by the electric power from the system, whereby the gas-turbine compressor is driven to feed the combustion air into the furnace. As the fuel is fed intothe furnace and the temperature and height of the fluidized bed increase, the boiler generates steam and hot gas from the burning coal. In this process of a load increase, the gas turbine generator switches from the synchronous motor to a synchronous generator.Change in Plant LoadIn a PFBC boiler, both the bed temperature and the main-steam temperature change according to the change in the fuel-feed rate. Therefore, to protect the equipment, it is necessary to maintain both temperatures within a certain range on a stable basis. In the present boiler, the bed height and the fuelfeed rate are adjusted to enable stable control of the bed and main-steam temperatures. When the bed height is changed, the area of contact between the heat exchanger and fluidized bed in the furnace changes, causing the quantity of the heat transferred from the furnace to the steam to change. As a result, both the bed temperature and the steam temperature change. Therefore, a PFBC plant needs a control system capable of keeping the bed and main-steam temperatures within the prescribed limits by controlling the fuel-feed rate and the bed height simultaneously. The present PFBC plant uses a system that controls the main steam temperature by the fuelfeed rate, which enables comparatively good plant operability and bed-temperature control with a comparatively large tolerance to the bed height. The measured characteristics of the plant behavior during a load change are shown in Fig. 7. CONCLUSIONSWe described the equipment of 250-MW Series 1,Unit 1-1 that was delivered to Osaki Power Station of The Chugoku Electric Power Co., Inc., as well as the results of the test operation. The PFBC plant went through the test operation smoothly, satisfactorily, demonstrating the planned performance. We intend to test the reliability of the PFBCequipment on the basis of operation results, which will be accumulated in the future. In concluding this paper, we would like to thank all the people of The Chugoku Electric Power Co., Inc., for their generous and extensive guidance and cooperation in all phases of the present PFBC project for Osaki Power Station, from plant planning to plant construction and test operation.REFERENCES(1)K. Horiuchi et al., “Test Operation of Osaki PFBC System,” The 7th National Symposium on Power and Energy Systems (Oct. 2000) in Japanese.(2) H. Komatsu et al., “Completion of a Large-Capacity Pressurized Fluidized Bed Combustion Boiler Combined Cycle Power Plant,” Hitachi Hyoron 83, pp.41-44 (Feb. 2001) inJapanese.(3) O. Ito et al., “Direct Measurement of Particle Motion on a Large-Scale FBC Boiler Model,” Proceedings of the 15th International Conference on Fluidized Bed Combustion, FBC99-0023 (May 1999).ABOUT THE AUTHORSHideaki Komatsu Joined Hitachi, Ltd. in 1974, and now works at the Thermal Power Plant Engineering Department of the Power & Industrial Systems. He is currently engagedin the development of thermal power plant systems. Mr. Komatsu is a member of Japan Society ofMechanical Engineers.Masakatsu Maeda Joined Babcock-Hitachi K.K. in 1965, and now works at the Thermal Power Division. He is currently engaged in the development of thermal power boilers.Mr. .Masaru Muramatsu Joined Hitachi, Ltd. in 1965 and now works atthe Power Plant Control Systems Engineering Department of the Power & Industrial Systems. He is currently engaged in the development of thermal power plant control systems.。
锅炉英语对照(共38页)
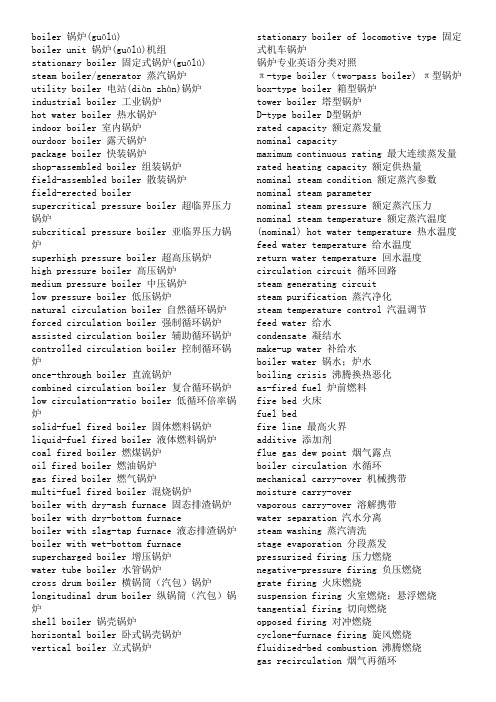
boiler 锅炉(guōlú)boiler unit 锅炉(guōlú)机组stationary boiler 固定式锅炉(guōlú) steam boiler/generator 蒸汽锅炉utility boiler 电站(diàn zhàn)锅炉industrial boiler 工业锅炉hot water boiler 热水锅炉indoor boiler 室内锅炉ourdoor boiler 露天锅炉package boiler 快装锅炉shop-assembled boiler 组装锅炉field-assembled boiler 散装锅炉field-erected boilersupercritical pressure boiler 超临界压力锅炉subcritical pressure boiler 亚临界压力锅炉superhigh pressure boiler 超高压锅炉high pressure boiler 高压锅炉medium pressure boiler 中压锅炉low pressure boiler 低压锅炉natural circulation boiler 自然循环锅炉forced circulation boiler 强制循环锅炉assisted circulation boiler 辅助循环锅炉controlled circulation boiler 控制循环锅炉once-through boiler 直流锅炉combined circulation boiler 复合循环锅炉low circulation-ratio boiler 低循环倍率锅炉solid-fuel fired boiler 固体燃料锅炉liquid-fuel fired boiler 液体燃料锅炉coal fired boiler 燃煤锅炉oil fired boiler 燃油锅炉gas fired boiler 燃气锅炉multi-fuel fired boiler 混烧锅炉boiler with dry-ash furnace 固态排渣锅炉boiler with dry-bottom furnaceboiler with slag-tap furnace 液态排渣锅炉boiler with wet-bottom furnace supercharged boiler 增压锅炉water tube boiler 水管锅炉cross drum boiler 横锅筒(汽包)锅炉longitudinal drum boiler 纵锅筒(汽包)锅炉shell boiler 锅壳锅炉horizontal boiler 卧式锅壳锅炉vertical boiler 立式锅炉stationary boiler of locomotive type 固定式机车锅炉锅炉专业英语分类对照π-type boiler(two-pass boiler) π型锅炉box-type boiler 箱型锅炉tower boiler 塔型锅炉D-type boiler D型锅炉rated capacity 额定蒸发量nominal capacitymaximum continuous rating 最大连续蒸发量rated heating capacity 额定供热量nominal steam condition 额定蒸汽参数nominal steam parameternominal steam pressure 额定蒸汽压力nominal steam temperature 额定蒸汽温度(nominal) hot water temperature 热水温度feed water temperature 给水温度return water temperature 回水温度circulation circuit 循环回路steam generating circuitsteam purification 蒸汽净化steam temperature control 汽温调节feed water 给水condensate 凝结水make-up water 补给水boiler water 锅水;炉水boiling crisis 沸腾换热恶化as-fired fuel 炉前燃料fire bed 火床fuel bedfire line 最高火界additive 添加剂flue gas dew point 烟气露点boiler circulation 水循环mechanical carry-over 机械携带moisture carry-overvaporous carry-over 溶解携带water separation 汽水分离steam washing 蒸汽清洗stage evaporation 分段蒸发pressurized firing 压力燃烧negative-pressure firing 负压燃烧grate firing 火床燃烧suspension firing 火室燃烧;悬浮燃烧tangential firing 切向燃烧opposed firing 对冲燃烧cyclone-furnace firing 旋风燃烧fluidized-bed combustion 沸腾燃烧gas recirculation 烟气再循环natural draft 自然通风mechanical draft 机械(jīxiè)通风balanced draft 平衡(pínghéng)通风forced draft 正压通风(tōng fēng)induced draft 负压(fù yā)通风zone control 分段送风pressure atomization 压力雾化;机械雾化mechanical atomizationtwin-fluid atomization 双流体雾化rotary-cup atomization 旋杯雾化;转杯雾化direct leakage 直接泄漏infiltration leakagebypass leakage 间接泄漏entrained leakageboiler proper 锅炉本体heating surface 受热面radiant heating surface 辐射受热面convection heating surface 对流受热面pressure part 受压部件;受压元件cylindrical shell 筒体head 封头;端盖header 集箱;联箱tube panel 管屏up flow riser tube panel 垂直上升管屏ribbon panel 回带管屏spirally-wound tubes 水平围绕管圈tube bundle 管束gas pass 烟道gas ductconvection pass 对流烟道parallel gas passes 并联烟道air duct 风道arch 拱furnace arch 折焰角water-cooled hopper bottom 冷灰斗wall with refractory lining 卫燃带refractory beltsupporting tube 悬吊管design pressure 设计压力maximum allowable working pressure 最高允许工作压力maximum allowable metal temperature 最高许用壁温furnace enclosure design pressure 炉膛设计压力heat input 输入热量heat output 锅炉有效利用热量fuel consumption 燃料消耗量calculated fuel consumption 计算燃料消耗量ash-retention efficiency 排渣率load range at constant temperature (保持)额定汽温的负荷范围injection flow(rate) 喷水量blowdown flow(rate) 排污量theoretical air 理论空气量excess air ratio 过量空气系数hot air temperature 热风温度exhaust gas temperature 排烟温度theoretical combustion temperature 理论燃烧温度adiabatic temperaturefurnace outlet gas temperature 炉膛出口烟气温度furnace exit gas temperaturepressure drop 汽水阻力draft loss 通风阻力pressure dropstack draft 自生通风压头available static head 运动压头circulation ratio 循环倍率circulation velocity 循环水速steam quality by mass 质量含汽率;干度mass velocity 质量流速critical steam quality 临界含汽率steam quality at minimum heat transfer coefficient 最高壁温处含汽率furnace volume 炉膛容积furnace volume heat release rate 炉膛容积热负荷heat liberation rate in furnacefurnace cross-section heat release rate 炉膛截面积热负荷furnace plan heat release rateburner zone wall heat release rate 燃烧器区域炉壁热负荷furnace wall heat release rate 炉壁热负荷furnace wall heat flux density 炉壁热流密度critical heat flux density 临界热流密度grate heat release rate 炉排(面积)热负荷burner heat input 燃烧器热功率ignition energy 点火能量evaporation rate 受热面蒸发率percentage of economizer evaporation 省煤器沸腾率primary air 一次风secondary air 二次风tertiary air 三次(sān cì)风imaginary circle 假想(jiǎxiǎng)切圆percentage of air space 通风(tōng fēng)截面比fineness 煤粉细度explosion mixture limits 爆炸(bàozhà)界限furnace 炉膛;炉胆fire box 火箱smoke box 烟箱burner 燃烧器tilting burner 摆动式燃烧器igniter 点火器oil atomizer 油雾化器register 调风器stabilizer 稳燃器wind box 风箱burner port 燃烧器喷口burner quarlgrate 炉排hand-fired grate 手烧炉排stoker-fired grate 机械炉排mechanical stokertravelling grate stoker 链条炉排chain grate stoker 链带式炉排bar grate stoker 横梁式炉排louvre stoker 鳞片式炉排vibrating stoker 振动炉排inclined reciprocating grate 往复炉排spreader stoker 抛煤机air compartment 风室reinjection system 飞灰复燃装置drum 锅筒;汽包steam drum 上锅筒water drum 下锅筒shell 锅壳drum internals 锅筒内部装置;汽包内部装置steam washer 清洗装置cyclone separator 旋风分离器turbo separator 轴流式分离器baffle plate 缝隙挡板corrugated scrubber 百叶窗分离器screen separator 钢丝网分离器perforated distribution plate 多孔板dry pipe 集汽管evaporating heating surface 蒸发受热面water-cooled wall 水冷壁membrane wall 膜式水冷壁division wall 双面水冷壁anti-clinker box 防焦箱gererating tube bank 锅炉管束boiler convection tube bankboiler (slag) screen 防渣管fire tube;smoke tube 烟管;火管mixer 混合器superheater 过热器radiant superheater 辐射过热器wall superheater 墙式过热器platen superheater 屏式过热器convection surperheater 对流过热器steam-cooled wall 包墙过热器steam-cooled roof 顶棚管过热器reheater 再热器attemperator 减温器desuperheatersurface type attemperator 面式减温器surface type desuperheaterspray type attemperator 喷水减温器spray type desuperheaterbiflux 汽-汽热交换器reheater superheater attemperator bypass damper 旁路挡板economizer 省煤器steaming economizer 沸腾式省煤器steel tube economizer 钢管省煤器finned tube economizer 鳍片管省煤器cast-iron gilled tube economizer 铸铁省煤器air heater 空气预热器tubular air heater 管式空气预热器rotary air heater 回转式空气预热器regenerative air heaterrotating-plate type regenerative air heater 受热面回转式预热器Ljungstrom type air heaterstationary-plate type regenerative air heater 风罩回转式预热器rothemuhle type air heatersteam air heater 暖风器boiler structure 锅炉构架top-supported structure by beams 支承式锅炉构架top-supported structure by hangers 悬吊式锅炉构架buckstay 刚性梁inner casing 内护板outer casing 外护板boiler steam and water circuit 锅炉(guōlú)汽水系统boiler external piping 锅炉(guōlú)范围内管道start-up system 启动(qǐdòng)系统start-up flash tank 启动(qǐdòng)分离器safety valve 安全阀safety relief valve 安全泄放阀water level indicator 水位表injector 注水器boiler setting 炉墙soot blower 吹灰器slag removal equipment 除渣设备boiler efficiency 锅炉效率;锅炉热效率boiler operating availability 锅炉可用率boiler forced outage rate 锅炉事故率feed water condition 给水品质steam purity 蒸汽品质moisture in steam 蒸汽湿度boiler water concentration 锅水浓度;炉水浓度total dissolved salt 总含盐量total solid (matter) 全固形物dissolved solid (matter) 溶解固形物suspended solid (matter) 悬浮物total hardness (总)硬度alkalinity 碱度heat loss 热损失heat loss due to exhaust gas 排烟热损失heat loss due to unburned gases 气体(化学)未完全燃烧热损失heat loss due to unburned carbon inrefuse 固体(机械)未完全燃烧热损失heat loss due to radiation 散热损失heat loss due to sensible heat in slag 灰渣物理热损失unburned combustible in flue dust 飞灰可燃物含量;飞灰含碳量unburned carbon in flue dustunburned combustible in slag 炉渣可燃物含量;炉渣含碳量unburned carbon in slagunburned combustible in sifting 漏煤可燃物含量dust loading 烟气含尘量dust densityload range of boiler 锅炉负荷调节范围turndown ratio 燃烧器调节比air leakage factor 漏风系数set pressure 整定压力start-to-discharge pressure 前泄压力popping pressure 起座压力reseating pressure 回座压力blowdown 回座压差discharge capacity 排放量;排汽能力boiler efficiency test 锅炉效率试验;锅炉热效率试验hydrostatic test 水压试验hydrostatic deformation test 验证性水压试验air leakage test 漏风试验pressure decay test 风压试验load test 负荷试验circulation test 水循环试验thermal chemical test 热化学试验sounding of tube by balls 通球试验safety valve operating test 安全阀校验flue gas analysis 烟气分析Orsat (gas analyser) 奥氏(烟气)分析器suction pyrometer 抽气式热电偶(高温计)venturi pneumatic pyrometer 气力式高温计heat flux meter 热流计start-up 启动filling 上水water level 水位initial water level 点火水位purge 吹扫blowoff 放水drain 疏水blowdown 排污raising pressure 升压bringing a boiler onto the line 并汽start-up pressure 启动压力start-up flow rate 启动流量shutdown 停炉outageout of service 停用banking fire 压火storage 停炉保护chemical cleaning 化学清洗boiling-out (碱)煮炉flushing 冲管steam-line blowing 吹管passivating 钝化drying-out 烘炉flow stagnation 停滞flow reversal 倒流separation of two-phase fluid 汽水分层steam binding 汽塞steam blanketingpriming 汽水(qìshuǐ)共腾foaming 泡沫(pàomò)共腾external deposit 烟气(yān qì)侧沉积物internal deposit 汽水(qìshuǐ)侧沉积物slagging 结渣fouling 积灰clogging 堵灰pitting attack 点状腐蚀ductile gouging 延性腐蚀hydrogen damage 氢脆caustic embrittlement 苛性脆化high temperature corrosion 高温腐蚀low temperature corrosion 低温腐蚀overheating 超温;过热flashback 回火blow off 脱火loss of ignition 熄火;灭火furnace explosion 炉膛爆炸furnace implosion 炉膛内爆furnace puff 炉膛爆燃blow hole 火口secondary combustion 二次燃烧1.parameter/参数rated capacity 额定蒸发量nominal capacitymaximum continuous rating 最大连续蒸发量rated heating capacity 额定供热量nominal steam condition 额定蒸汽参数nominal steam parameternominal steam pressure 额定蒸汽压力nominal steam temperature 额定蒸汽温度(nominal)hot water temperature 热水温度feed water temperature 给水温度return water temperature 回水温度circulation circuit 循环回路steam generating circuitsteam purification 蒸汽净化steam temperature control 汽温调节feed water 给水condensate 凝结水make-up water 补给水boiler water 锅水,炉水boiling crisis 沸腾换热恶化as-fired fuel 炉前燃料fire bed,fuel bed 火床fire line 最高火界additive 添加剂flue fas dew point 烟气露点Components for CFBC boiler (CFBC锅炉的主要部件)2.基本工作原理boiler circulation 水循环mechanical carry - over 机械携带moisture carry - overvaporous carry - over 溶解携带water separation 汽水分离steam washing 蒸汽清洗stage evaporation 分段蒸发pressurized firing 压力燃烧negative - pressure firing 负压燃烧grate firing 火床燃烧suspension firing 火室燃烧,悬浮燃烧tangential firing 切向燃烧opposed firing 对冲燃烧cyclone - furnace firing 旋风燃烧fluidized - bed combustion 沸腾燃烧gas recirculation 烟气再循环natural draft 自然通风mechanical draft 机械通风balanced draft 平衡通风forced draft 正压通风induced draft 负压通风zone control 分段送风pressure atomization 压力雾化,机械雾化mechanical atomizationtwin-fluid atomization 双流体雾化rotary-cup atomization 旋杯雾化,转杯雾化direct leakage 直接泄漏infiltration leakagebypass leakage 间接泄漏entrained leakage3.structure/结构boiler proper 锅炉本体heating surface 受热面radiant heating surface 辐射受热面convection heating surface 对流受热面pressure part 受压部件/元件cylidrical shell 筒体head(drum)封头head(heater)端盖header 集箱tube panel 管屏up flow riser tube panel 垂直上升管屏ribbon panel 回带管屏spirally - wound tubes 水平围绕管圈tube bundle 管束gas pass ,gas duct 烟道炉内/炉外convection pass 对流(duìliú)烟道parallel gas pass 并联(bìnglián)烟道air duct 风道(fēnɡ dào)arch 拱furnace arch 折焰角water - cooled hopper bottom 冷灰斗wall with refractory lining 卫燃带refractory beltsupporting tube 悬吊管4.设计参数(cānshù)和指标design pressure 设计压力maximum allowable working pressure 最高允许工作压力maximum allowable metal temperature 最高许用壁温furnace enclosure design pressure 炉膛设计压力heat input 输入热量heat output 锅炉有效利用热量fuel consumption 燃料消耗量calculated fuel consumption 计算燃料消耗量ash-retention efficiency 排渣率额定汽温的负荷范围injection flow(rate) 喷水量blowdown flow(rate) 排污量theoretical air 理论空气量excess air ratio 过量空气系数hot air tempereture 热风温度exhaust gas tempereture 排烟温度theoretical combustion tempereture 理论燃烧温度adiabatic tempereturefurnace outlet gas tempereture 炉膛出口烟气温度funace exit gas tempereturepressure drop 汽水阻力draft loss (用于负压燃烧)通风阻力pressure drop(压力燃烧)stack draft 自生通风压头available static head 运动压头circulation ratio 循环倍率circulation velocity 循环水速steam quality by mass 质量含汽率,干度mass velocity 质量流速critical steam quality 临界含汽率steam quality at minimum heat transfer coefficient 最高壁温处含汽率furnace volume 炉膛容积furnace volume heat release rate 炉膛容积热负荷heat liberation rate in furnacefurnace cross-section heat release 炉膛截面积热负荷furnace plan heat release rateburner zone wall heat release rate 燃烧器区域热负荷furnace wall heat release rate 炉壁热负荷fernace wall heat flux density 炉壁热流密度critical heat flux density 临界热流密度grate heat release rate 炉排(面积)热负荷burner heat input 燃烧器热功率ignition energy 点火能量evaporation rate 受热面蒸发率percentage of economizer evaporation 省煤器沸腾率Primary air 一次风Secondary air 二次风imaginary circle 假想切圆percentage of air space 通风截面积fineness 煤粉细度explosion mixture limits 爆炸界限5.主要零部件,元件和附件炉膛和燃烧设备furnace 炉膛fire box 火箱smoke box 烟箱burner 燃烧器tilting burner 摆动式燃烧器igniter 点火器oil atomizer 油雾化器register 调风器stabilizer 稳燃器Wind box 风箱burner port/burner fquarrel 燃烧器喷口grate 炉排inclined reciprocating grate 往复炉排pnleum 风室6.drum & drum internal 锅筒及其内部装置drum 锅筒steam drum 上锅筒/汽包water drum 下锅筒shell 锅壳drum linternals 锅筒/汽包内部装置steam washer 清洗装置cyclone scrubber 旋风分离器turbo separator 轴流式分离器baffle plate 波形板coffugated scrubbber 百叶窗分离器screen separator 钢丝(ɡānɡ sī)分离器perforated distribution plate 多孔板dry pipe 集汽管7.evaporating heating surface/蒸发(zhēngfā)受热面evaporating heating surface 蒸发(zhēngfā)受热面water-cooled wall 水冷壁membrane wall 膜式水冷壁division wall 双面水冷壁anti -clinker box 防焦箱generating tube bank 锅炉(guōlú)管束boiler convection tube bank 锅炉管束boiler (slag) screen 放渣管smoke tube 火管mixer 混合器8.superheater &reheater/ 过热器及再热器superheater 过热器radiant superheater 辐射过热器wall superheater 包墙过热器platen superheater 屏式过热器convection superheater 对流过热器steam-cooled wall 汽冷包墙steam -cooled roof 汽冷顶棚reheater 再热器attemperator 减温器surface type attemperator 面式减温器Spray type attemperator 喷水减温器bypass damper 旁路挡板biflux 汽-汽热交换器9.economizer 省煤器economizer 省煤器steam economizer 沸腾式省煤器steel tube economizer 钢管省煤器finned tube economizer 鳍片省煤器cast-lron economizer 铸铁省煤器10.air heater / 空气预热器air heater 空气预热器tubular air heater 管式空气预热器steam air heater 暖风管11.structure / 构架boiler structure 锅炉构架top-supported struture by beams 支撑式锅炉构架top -supported structure by hangers 悬吊式锅炉构架buckstay 刚性梁inner casing 内护板outer casing 外护板12.piping & accessory / 管道及附件boiler steam and water circuit 锅炉汽水系统boiler external piping 锅炉范围内管道start-up system 启动系统start-up flash tank 启动排污罐safety valve 安全阀safety relief valve 安全泄放阀water level indicator 水位表injector 注水器level switch 电接点水位计13.Others 其他boiler setting 炉墙soot blower 吹灰器slag removal equipment 除渣设备14.technic index/ 技术指标boiler efficiency 锅炉效率(热效率)boiler operating availability 锅炉可用率boiler forced outage rate 锅炉事故率feed water condition 给水品质steam purity 蒸汽品质moisture in steam 蒸汽湿度boiler water concentration 锅水浓度,炉水浓度total dissolved salt 总含盐量tatal solid(matter)全固形物dissolved solid(matter) 溶解固形物suspended solid(matter) 悬浮物tatal hardness (总)硬度alkalinity 碱度heat loss 热损失heat loss due to exhaust gas 排烟热损失heat loss due to unburned gases 气体(化学)未完全燃烧热损失heat loss due to unburned carbon in refuse 固体(机械)未完全燃烧热损失heat loss due to flue dust 散热损失heat loss due to sensible heat in slag 灰渣物理热损失undurned combustible in flue dust 飞灰可燃物含量undurned cabon in flue dust 飞灰含碳量undurned cabon in slag 炉渣含碳量undurned combustible in sifting 漏煤可燃物含量dust loading/dust density 烟气含尘量load range of bioler 锅炉负荷调节范围turndown ratio 燃烧器调节比air leakage factor 漏风(lòu fēng)系数set pressure 整定压力(yālì)start-to-discharge pressure 前泄压力(yālì) popping pressure 起座压力(yālì)reseating pressure 回座压力blowdown 回座压差discharge capacity 排放量。
锅炉系统毕业设计论文中英文资料对照外文翻译文献
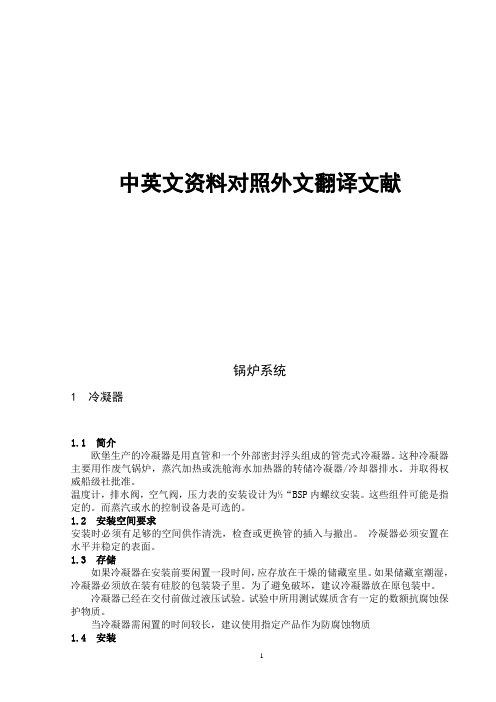
中英文资料对照外文翻译文献锅炉系统1 冷凝器1.1 简介欧堡生产的冷凝器是用直管和一个外部密封浮头组成的管壳式冷凝器。
这种冷凝器主要用作废气锅炉,蒸汽加热或洗舱海水加热器的转储冷凝器/冷却器排水。
并取得权威船级社批准。
温度计,排水阀,空气阀,压力表的安装设计为½“BSP内螺纹安装。
这些组件可能是指定的。
而蒸汽或水的控制设备是可选的。
1.2 安装空间要求安装时必须有足够的空间供作清洗,检查或更换管的插入与撤出。
冷凝器必须安置在水平并稳定的表面。
1.3 存储如果冷凝器在安装前要闲置一段时间,应存放在干燥的储藏室里。
如果储藏室潮湿,冷凝器必须放在装有硅胶的包装袋子里。
为了避免破坏,建议冷凝器放在原包装中。
冷凝器已经在交付前做过液压试验。
试验中所用测试媒质含有一定的数额抗腐蚀保护物质。
当冷凝器需闲置的时间较长,建议使用指定产品作为防腐蚀物质1.4 安装冷凝器设计为垂直或水平安装。
在水平安装的情况下,蒸汽喷嘴必须朝上,而冷凝水出口喷嘴朝下。
如果是垂直安装冷凝器,蒸汽入口和海水出口端必须朝上。
排水和空气排放阀必须安装在冷凝器在最低和最高点的中间线的位置。
任何选择性的控制设备必须根据具体指示安装。
步骤A:将冷凝器安装在水平平面上。
步骤B:钻基础固定螺栓孔。
步骤C:将螺栓放入孔中并拧紧。
连接冷凝器步骤D:移除所有的塞子和盲板,然后再连接冷凝器。
步骤E:在连接中确保没有杂质进入。
步骤F:管道连接起来,确保从管道和冷凝器之间没有强制力的产生。
1.5 调试启动前要确保所有连接都牢固地拧紧是很重要的。
同样重要的是,冷凝器和连接管道空气要彻底排出。
步骤A:如果装有安全阀,必须加以调整到最大设计压力或较低。
步骤B:法兰螺栓要拧紧。
拧紧法兰螺栓时始终使用扭矩扳手。
步骤C:运行一小时,停止冷凝器,并重新拧紧所有螺栓。
步骤D:启动阶段,冷凝器的两边都要排出空气,必须认真仔细的检查回路的泄漏。
1.6 性能冷凝器性能须附和传热计算表规定的要求。
燃煤锅炉 外文翻译 外文文献 英文文献 中英翻译

燃煤锅炉外文翻译外文文献英文文献中英翻译外文出处: A. A. Shatil’, N. S. K. A. A. S., & V. Kudryavtsev, A. (2008). Controllingthe furnace process in coal-fired boilers. Thermal Engineering, 55, 1, 72-77.Controlling the Furnace Process in Coal-Fired BoilersThe unstable trends that exist in the market of fuel supplied to thermal power plants and the situations in which the parameters of their operation need to be changed (or preserved), as well as the tendency toward the economical and environmental requirements placed on them becoming more stringent, are factors that make the problem ofcontrolling the combustion and heat transfer processes in furnace devices very urgent. The solution to this problem has two aspects. The first involves development of a combustion technology and,accordingly, the design of a furnace device when new installations are designed. The second involves modernization of already existing equipment. In both cases,the technical solutions being adopted must be properly substantiated with the use of both experimental and calculationstudies.The experience Central Boiler-Turbine Institute Research and Production Association (TsKTI) and ZiO specialists gained from operation of boilers and experimental investigations they carried out on models allowed them to propose several new designs of multifuel andmaneuverable—in other words, controllable—furnace devices that had been put in operationat power stations for several years. Along with this, an approximate zero-one-dimensional, zonewise calculation model of the furnace process in boilers had been developed at the TsKTI, which allowed TsKTI specialists to carry out engineering calculations of the main parameters of this process and calculate studies of furnaces employing different technologies of firing and combustion modes .Naturally, furnace process adjustment methods like changing the air excess factor, stack gas recirculation fraction, and distribution offuel and air among the tiers of burners, as well as other operations written in the boiler operational chart, are used during boiler operation.However, the effect they have on the process is limited in nature. On the other hand, control of the furnace process in a boiler implies the possibility of making substantial changes in the conditions under which the combustion and heat transfer proceed in order to considerably expand the range of loads, minimize heat losses, reduce the extent to which the furnace is contaminated with slag, decrease the emissions of harmful substances, and shift to another fuel. Such a control can be obtained by making use of the following three main factors:(i) the flows of oxidizer and gases being set to move in the flamein a desired aerodynamic manner;1(ii) the method used to supply fuel into the furnace and the placeat which it is admitted thereto;(iii) the fineness to which the fuel is milled.The latter case implies that a flame-bed method is used along withthe flame method for combusting fuel.The bed combustion method can be implemented in three design versions: mechanical grates with a dense bed, fluidized-bed furnaces, and spouted-bed furnaces.As will be shown below, the first factor can be made to work bysetting up bulky vortices transferring large volumes of air and combustion products across and along the furnace device. If fuel isfired in a flame, the optimal method of feeding it to the furnace is to admit it to the zones near the centers of circulating vortices, a situation especially typical of highly intense furnace devices. The combustion process in the se zones features a low air excess factor (α< 1) and a long local time for which the components dwell in them, factors that help make the combustion process more stable and reduce theemission of nitrogen oxides .Also important for the control of a furnace process when solid fuelis fired is the fineness to which it is milled; if we wish to minimize incomplete combustion, the degree to which fuel is milled should be harmonized with the location at which the fuel is admitted into the furnace and the method for supplying it there, for the occurrence of unburned carbon may be due not only to incomplete combustion of large-size fuel fractions, but also due to fine ones failing to ignite (especially when the content of volatiles Vdaf < 20%).Owing to the possibility of pictorially demonstrating the motion of flows, furnace aerodynamics is attracting a great deal of attention of researchers and designers who develop and improve furnace devices. At the same time, furnace aerodynamics lies at the heart of mixing (mass transfer), a process the quantitative parameters of which can be estimated only indirectly or by special measurements. The quality with which components are mixed in the furnace chamber proper depends on the number, layout, and momenta of the jets flowing out from individual burners or nozzles, as well as on their interaction with the flow of flue gases, with one another, or with the wall.It was suggested that the gas-jet throw distance be used as a parameter determining the degree to which fuel is mixed with air in the gas burner channel. Such an approach to estimating how efficient the mixing is may to a certain degree be used in analyzing the furnace as a mixing apparatus. Obviously, the greater the jet length (and its momentum), the longer the time during which the velocity gradient it creates in the furnace will persist there, a parameter that determines how completely the flows are mixed in it. Note that the higher the degree to which a jet is turbulized at the outlet from a nozzle or burner, the shorter the distance which it covers, and, accordingly, the less completely the components are mixed in2the furnace volume. Once through burners have advantages over swirl ones in this respect.It is was proposed that the extent to which once through jets are mixed as they penetrate with velocity w2 and density ρ2 into a transverse (drift) flow moving with velocity w1 and having density ρ1 be correlated with the relative jet throw distance in the following way Where ks is a proportionality factor tha t depends on the ―pitch‖ between the jet axes (ks=1.5–1.8).The results of an experimental investigation inwhich the mixing of gas with air in a burner and then in a furnace was studied using the incompleteness of mixing as a parameter are reported in 5.A round once through jet is intensively mixed with the surrounding medium in a furnace within its initial section, where the flow velocity at the jet axis is still equal to the velocity w2 at the nozzle orifice of radius r0.The velocity of the jet blown into the furnace drops very rapidly beyond the confines of the initial section, and the axis it has in the case of wall-mounted burners bends toward the outlet from the furnace.One may consider that there are three theoretical models for analyzing the mixing of jets with flowrate G2 that enter into a stream with flowrate G1. The first model is for the case when jets flow into a ―free‖ space (G1= 0),the second model is for the case when jets flowinto a transverse (drift) current with flowrate G1G2,and the third model is for the case ,when jets flow into a drift stream with flowrate G1<G2. The second model represents mixing in the channel of a gas burner, and the third model represents mixing in a furnace chamber. We assume that the mixing pattern we have in a furnace is closer to the first model than it is to the second one, since 0 <G1/G2< 1, and we will assume that the throw distance h of the jet being drifted is equal to the length S0 of the―free‖ jet’s initial section. The ejection ability of the jet being drifted then remains the same as that of the ―free‖ jet, and the length of the initialsection can be determined using the well-known empirical formula of G.N. Abramovich [6] :S0= 0.67r0/a, (2)where a is the jet structure factor and r0 is the nozzle radius.At a = 0.07, the length of the round jet’s initial section is equal to 10 r0 and the radius the jet has at the transition section (at the end of the initial section) is equal to 3.3 r0. The mass flowrate in the jet is doubled in this case. The corresponding minimum furnace cross-sectional area Ff for a round once through burner with the outlet cross-sectional area Fb will then be equal to and the ratio Ff/Fb?20. This value is close to the actual values found in furnacesequipped with once through burners. In furnaces equipped with swirl burners, a= 0.14 and Ff/Fb?10. In both cases, the interval between theburners is equal to the jet diameter in the transition section d tr , which differs little from the value that has been established inpractice and recommended in [7].3The method traditionally used to control the furnace process inlarge boilers consists of equipping them with a large number of burners arranged in several tiers. Obviously, if the distance between the tiers is relatively small, operations on disconnecting or connecting themaffect the entire process only slightly. A furnace design employinglarge flat-flame burners equipped with means for controlling the flame core position using the aerodynamic principle is a step forward. Additional possibilities for controlling the process in TPE-214 and TPE-215 boilers with a steam output of 670 t/h were obtained through the use of flat-flame burners arranged in two tiers with a large distance between the tiers; this made it possible not only to raise or lower the flame, but also to concentrate or disperse the release of heat in it [1].A very tangible effect was obtained from installing multifuel (operating on coal and open-hearth, coke, and natural gases) flat-flame burners in the boilers of cogeneration stations at metallurgical plants in Ukraine and Russia.Unfortunately, we have to state that, even at present, those in charge of selecting the type, quantity, and layout of burners in a furnace sometimes adopt technical solutions that are far from being optimal. This problem should therefore be considered in more detail.If we increase the number of burners nb in a furnace while retaining their total cross-sectional area (ΣFb=idem) and the total flowrate ofair through them, their equivalent diameters deq will become smaller, as willthe jet momentums Gbwb, resulting in a corresponding decrease in the jet throw distance hb and the mass they eject. The space with high velocity gradients also becomes smaller, resulting in poorer mixing in the furnace as a whole. This factor becomes especially important whenthe emissions of NOx and CO are suppressed right inside the furnaceusing staged combustion (at αb < 1) under the conditionsof a fortiori nonuniform distribution of fuel among the burners.In [1], a quantitative relationship was established between the parameters characterizing the quality with which once through jets mix with one another as they flow into a limited space with the geometrical parameter of concentration = with nb = idem and Gb = idem. By decreasing this parameter we improve the mass transfer in the furnace; however,this entails an increase in the flow velocity and the expenditure of energy (pressure drop) in the burners with the same Fb. At the same time, we know from experience and calculations that good mixing in a furnace can be obtained without increasing the head loss if we resort to large long-range jets. This allows a much less stringent requirement to be placed on the degree of uniformity with which fuel must be distributed among the burners. Moreover, fuel may in this case be fed to the furnace location where it is required from process control considerations.For illustration purposes, we will estimate the effect the number of burners has on the mixing in a furnace at = = idem. schematically shows the plan views of two furnace chambers4differing in the number of once through round nozzles (two and four) placed in a tier (on one side of the furnace). The furnaces have the same total outlet cross-sectional areas of the nozzles (ΣFb) and the same jet velocities related to these areas (wb). The well-known swirl furnace of the TsKTI has a design close to the furnace arrangement under consideration. According to the data of [1], the air fraction βair that characterizes the mixing and entersthrough once through burners into the furnace volume beneath themcan be estimated using the formula βair = 1 – (3) which has been verified in the range = 0.03–0.06 for a furnacechamber equipped with two frontal once through burners. Obviously,if we increase the number of burners by a factor of 2, their equivalent diameter, the length of the initial section of jets S0 and the area they ―serve‖ will reduce by a factor of Then, for example, at = 0.05, the raction βair will decreas e from 0.75 to 0.65. Thus, Eq. (3) may be written in the following fform for approximately assessing the effect of once through burners on the quality of mixing in a furnace:βair = 1 – 3.5f nb ' ,where is the number of burners (or air nozzles) on one wall when they are arranged in one tier both in onesided and opposite manners.The number of burners may be tentatively related to the furnacedepth af (at the same = idem) using the expression (5)It should be noted that the axes of two large opposite air nozzles ( = 1)—an arrangementimplemented in an inverted furnace—had to be inclined downward by more than 50? [8].One well-known example of a furnace device in which once throughjets are used to create a large vortex covering a considerable part of its volume is a furnace with tangentially arranged burners. Such furnaces have received especially wide use in combination with pulverizing fans. However, burners with channels having a small equivalent diameter are frequently used for firing low-calorific brown coals with high content of moisture. As a result, the jets of air-dust mixture and secondary air that go out from their channels at different velocities(w2/w1 = 2–3) become turbulized and lose the ability to be thrown a long distance; as a consequence, the flame comes closer to the waterwalls and the latter are contaminated with slag. One method by which the tangential combustion scheme can be improved consists of organizing the so-called concentric admission of large jets of air-dust mixture and secondary air with the fuel and air nozzles spaced apart from one another over the furnace perimeter, accompanied by intensifying the ventilation of mills [9, 10]. Despite the fact that thetemperature level in the flame decreases, the combustion does not become less stable because the fuel mixes with air in a stepwise manner in a horizontal plane.Vortex furnace designs with large vortices the rotation axes ofwhich are arranged transversely with respect to the main direction of gas flow have wide possibilities in terms of5controlling the furnace process. In [1], four furnace schemes with a controllable flame are described, which employ the principle of large jets colliding with one another; three of these schemes have been implemented. A boiler with a steam capacity of 230 t/h has been retrofitted in accordance with one of these schemes (with an inverted furnace) . Tests of this boiler, during which air-dust mixture was fed at a velocity of 25–30 m/s from the boiler frontusing a highconcentration dust system, showed that the temperatureof gases at the outlet from the furnace had a fairly uniformdistribution both along the furnace width and depth . A simple method of shifting the flame core over the furnace height was checked during the operation of this boiler, which consisted of changing the ratio of air flowrates through the front and rear nozzles;this allowed a shift to be made from running the furnace in a dry-bottom mode to a slag-tap mode and vice versa. A bottom-blast furnace scheme has received rather wide use in boilers equipped with different types of burners and mills. Boilers with steam capacities ranging from 50 to 1650 t/h with such anaerodynamic scheme of furnaces manufactured by ZiO and Sibenergomash have been installed at a few power stations in Russia and abroad . We have to point out that, so far as the efficiency of furnace process control is concerned, a combination of the following two aerodynamic schemes is of special interest: the inverted scheme and the bottom-blast one. The flow pattern and a calculation analysis of the furnace process in such a furnace during the combustion of lean coal are presented in [13].Below, two other techniques for controlling the furnace process are considered. Boilers with flame–stoker furnaces have gained acceptancein industrial power engineering, devices that can be regarded to certain degree as controllable ones owing to the presence of two zones in them . Very different kinds of fuel can be jointly combusted in these furnaces rather easily. An example of calculating such a furnace device is given in [2]. As for boilers of larger capacity, work on developing controllable two-zone furnaces is progressing slowly . The development of a furnace device using the so-called VIR technology (thetransliterated abbreviation of the Russian introduction, innovation, and retrofitting) can be considered as holding promise in this respect. Those involved in bringing this technology to the state of industry standard encountered difficulties of an operational nature (the control of the process also presented certain difficulties). In our opinion, these difficulties are due to the fact that the distribution of fuel over fractions can be optimized to a limited extent and that the flow inthe main furnace volume has a rather sluggish aerodynamic structure. It should also be noted that the device for firing the coarsest fractions of solid fuel in a spouting bed under the cold funnel is far from being technically perfect.Centrifugal dust concentrators have received acceptance for firing high-reactive coals in schemes employing pulverizing fans to optimize the distribution of fuel as to its flowrate and6fractions. The design of one such device is schematically shown in [9]. Figure shows a distribution of fuel flowrates among four tiers of burners that is close to the optimum one. This distribution can be controlled if we furnish dust concentrators with a device with variable blades, a solution that has an adequate effect on the furnace process.7燃煤锅炉的燃烧进程控制存在于火电厂的市场的燃料供应,某些操作参数需要改变(或保留)的情况下,以及经济和环境方面倾向的要求使他们变得更加严格的不稳定趋势是导致使控制燃烧与传热过程炉设备非常紧迫的主要因素。
给煤机论文中英文资料外文翻译文献
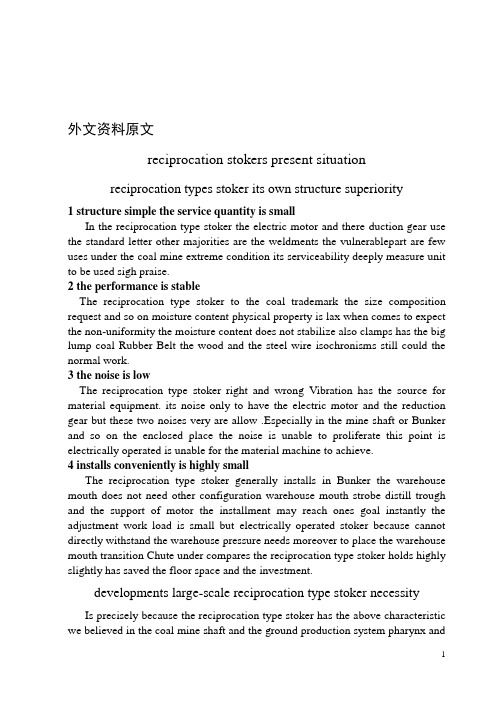
外文资料原文reciprocation stokers present situationreciprocation types stoker its own structure superiority1 structure simple the service quantity is smallIn the reciprocation type stoker the electric motor and there duction gear use the standard letter other majorities are the weldments the vulnerablepart are few uses under the coal mine extreme condition its serviceability deeply measure unit to be used sigh praise.2 the performance is stableThe reciprocation type stoker to the coal trademark the size composition request and so on moisture content physical property is lax when comes to expect the non-uniformity the moisture content does not stabilize also clamps has the big lump coal Rubber Belt the wood and the steel wire isochronisms still could the normal work.3 the noise is lowThe reciprocation type stoker right and wrong Vibration has the source for material equipment. its noise only to have the electric motor and the reduction gear but these two noises very are allow .Especially in the mine shaft or Bunker and so on the enclosed place the noise is unable to proliferate this point is electrically operated is unable for the material machine to achieve.4 installs conveniently is highly smallThe reciprocation type stoker generally installs in Bunker the warehouse mouth does not need other configuration warehouse mouth strobe distill trough and the support of motor the installment may reach ones goal instantly the adjustment work load is small but electrically operated stoker because cannot directly withstand the warehouse pressure needs moreover to place the warehouse mouth transition Chute under compares the reciprocation type stoker holds highly slightly has saved the floor space and the investment.developments large-scale reciprocation type stoker necessityIs precisely because the reciprocation type stoker has the above characteristic we believed in the coal mine shaft and the ground production system pharynx andlarynx link and in other need control noises links should the first choice reciprocation type stoker. The expanded series reciprocation type stoker should satisfy the following request.1 satisfies the large-scale mine pit productivity the requestAlong with the our country coal mine pit unceasing expansion the hour productivity also is increasing for example: The well is 2.4million t/a3 million t/a.4 XI ten thousand t/a mine pit hour productivity separately for 42 h 928t/h 1238t/h. The mine pit hour productivity increase the request enhances the stoker the productivity. At present the mine pit mine shaft raw coal transportation more and more many uses the adhesive tape conveyer in other words the mine shaft used the stoker the link to increase. Although may use many small models stoker jointly to arrange satisfies the big productivity the request but arranges many stokers to need to expand Weighed the room the increase project investment moreover many arrangement the system reliability reduces the noise increases has the problem the probability also relatively to increase. brings the certain trouble to the service. Loads car the system in the use adhesive tape conveyer is in the ground production system uses stoker most places moreover the request gives the coal ability quite to be big. Loads a car the system if uses Electric vibrator for the material machine not only increased has loaded a car moreover the noise very to be highly big. Installs the large-scale reciprocation type stoker not only causes the hour productivity to increase moreover also for loaded a car the system equipment shaping to provide has been bigger may choose the scope.2 loads the belt type necessary with the host well bottom of the wellbeat the conveyerThe main well bottom of the well loads the belt type beat the quota conveyer type bottom of the well charging gear development tendency lists as the coal key scientific research item when the quota conveyer idling speed loading is requested for the coal equipment ability 801 above ttb the existing K series stoker cannot meet this requirements. Although also may use for the material strobe material but for the material strobe for estimated easily the raw coal moisture the granularity influence causes for the material non-uniformity. But the large-scale reciprocation type stoker may satisfy this requestLarge-scale reciprocation types deceive the coal machine structuralstyle characteristicWe through carry on the analysis to the former stoker investigation has carried on the thorough computation to the structure pattern to the essential stress spot like tank the double connecting rod the base held the roller and so on to carryon the stress analysis carried on the examination computation using the finite element principle. Altogether has following five characteristics.1 entire machine uses the frameThemselves to be possible to unload the type Because considered the mine shaft transportation the convenience and the mine shaft industry and mining limit entire machine uses a minute writing style moreover considered the mineshaft welding inconvenient uses the bolt vice-joint the side bar to consider the external drum the possibility and the end ophragm wear the need lays down in the flank Reinforced inside Lining strengthened the whole rigidity and the intensity.2 increases Dao ban the guidance and the blockingIn makes the reciprocal motion under Dao ban to additionally build several groups of requests rollers wheel on the one hand changes Dao ban the sliding friction for to roll the friction reduced the attrition on the other hand also causes Dao ban the rigidity enhancement. Moreover for prevented Dao ban is transporting Moves in the process to have runs the leaning phenomenon in Dao ban two sides installments guidance wheel.3 uses the hyperbolic trough drive typeThe stoker drive uses the hard tooth face reduction gear or dinary plug shaft coupling but non-fluid strength coupler double output shaft form like this not only reduced the cost moreover to cause the unilateral Axis radial direction load only is the bottom surface thrust force one half is carried the condition big improvement moreover further enhanced the stability which Dao ban moved.4 carries on the optimization to the Dao ban structureFormerly Kseries stoker plate strobe the material had many problems in shipping for estimated could not enhance the key also lay in this. Therefore we have carried on the optimization to Dao ban designs for the steps and ladders Not only caused the material process load to enlarge more over the Dao ban itself intensity also obtained further enhances.5 changes the fan strobe for the manual plate strobeControl sexpects how many the key to be decided by the strobe the strobe always suses the fan structure in the former K series but discovered from the field use the fan strobe and the warehouse wall frequently bump the scene often when installs the strobe can not but knock the warehouse walla part causes the warehouse wall the intensity to weaken based on we uses the manual plate strobe matches the guidance wheel under the damper plate simultaneously has the positioning device on the axis.economic efficiency analysisIn needs 2000t/under the h transport capacity condition arranges twoUniversity of Taiwan stoker with simultaneously to arrange four K 14 stokers or four Electric vibrator stoker sits economic efficiency quite is as follows.1 Weighed proclaims the mining quantityThe big Weighed room not only causes the mining quantity to increase moreover can bring many negative effects along with it four reciprocation types stoker union arrangement should consider between the equipment must remain has the overhaul space holds Zuo the space like this its Weighed room resilience is bigger than two University of Taiwan reciprocation type stoker But if uses four vibrations to deceive the material mach ineneeds the height difference in a big way moreover because its noise is big the small Weighed room cannot satisfy its request. Speaking of this parameter arranges two University of Taiwan stoker the plan is most economical.2 power consumption comparisonThe large-scale reciprocation type stoker power is not certainly big two University of Taiwan stoker power consumption and four K 14 stokers or Electric vibrator the stoker is nearly impartial.3 equipment operating cost comparisonLarge-scale stoker vulnerable very few moreover because uses the standard hard tooth face reduction gear causes the service quantity minimum but four stokers because number increasing the maintenance cost also is corresponding is large-scale stoker two times Electric vibrator the stoker is unable in this point with it to compare. Develops the large-scale reciprocation type stoker met our country coal need to develop expanded in the coal mine production system design to give the coal equipment the shaping scope. In particular in Jining two mine pits success uses for me The country provided has been new large-scale for the coal equipment should give the vigorously promoted use.中文译文往复给煤机现状一、往复式给煤机其自身结构的优越性1 结构简单,维修量小在往复式给煤机中,电动机和减速器均采用标准件,其余大部分是焊接件,不易损坏,在煤矿井下这种恶劣的条件下,它的适应能力较好,具有通用性,受到广泛喜爱。
直流锅炉的湿干态运行-中英文
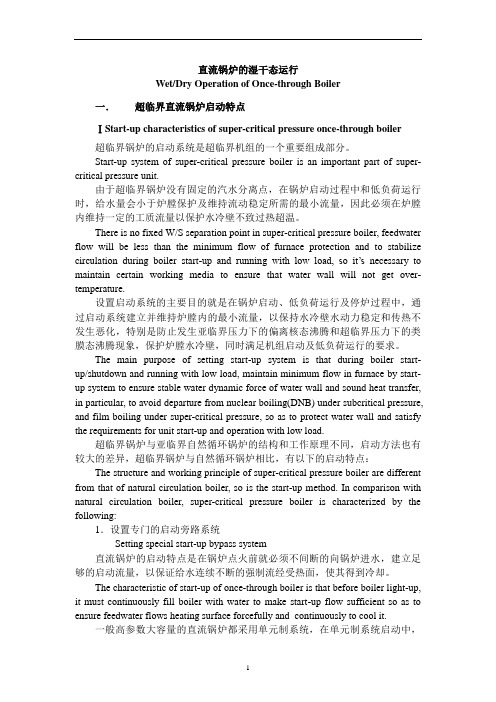
直流锅炉的湿干态运行Wet/Dry Operation of Once-through Boiler一.超临界直流锅炉启动特点ⅠStart-up characteristics of super-critical pressure once-through boiler超临界锅炉的启动系统是超临界机组的一个重要组成部分。
Start-up system of super-critical pressure boiler is an important part of super-critical pressure unit.由于超临界锅炉没有固定的汽水分离点,在锅炉启动过程中和低负荷运行时,给水量会小于炉膛保护及维持流动稳定所需的最小流量,因此必须在炉膛内维持一定的工质流量以保护水冷壁不致过热超温。
There is no fixed W/S separation point in super-critical pressure boiler, feedwater flow will be less than the minimum flow of furnace protection and to stabilize circulation during boiler start-up and running with low load, so it’s necessary to maintain certain working media to ensure that water wall will not get over-temperature.设置启动系统的主要目的就是在锅炉启动、低负荷运行及停炉过程中,通过启动系统建立并维持炉膛内的最小流量,以保持水冷壁水动力稳定和传热不发生恶化,特别是防止发生亚临界压力下的偏离核态沸腾和超临界压力下的类膜态沸腾现象,保护炉膛水冷壁,同时满足机组启动及低负荷运行的要求。
- 1、下载文档前请自行甄别文档内容的完整性,平台不提供额外的编辑、内容补充、找答案等附加服务。
- 2、"仅部分预览"的文档,不可在线预览部分如存在完整性等问题,可反馈申请退款(可完整预览的文档不适用该条件!)。
- 3、如文档侵犯您的权益,请联系客服反馈,我们会尽快为您处理(人工客服工作时间:9:00-18:30)。
Abstract In this paper, a practical method to design a robust controller for pressure of boiler in Mashhad Power plant using H∞ is proposed. To achieve a good performance of an industrial boiler in order to reduce fuel consumption rate and reaching higher efficiency, dynamic variables such as fuel flow, air flow and pressure of boiler must be controlled. However this dynamic model may associate with uncertainties. Uncertainties in mentioned model are caused by lack of knowledge about the dynamics of the system, pay load changes, and air flow. Thus, application of robust control methods for high precise control of pressure is inevitable. In the first step plant is identified by using experimental data by the mean of converting into a group of linear time invariant (LTI) uncertain plants. After representation of the uncertain dynamic system in general control configuration and modelling the parametric uncertainties, nominal performance, robust stability and robust performance against disturbances are analyzed by the concept of structured singular value µ. Then, using genetic algorithm an optimal controller is designed for tracking problem and disturbance rejection. This procedure gives a satisfactory controller at the presence of model perturbations. For more comparisons, quantitative feedback theory (QFT) as a well known robust control approach is also applied to the plant. Finally, nonlinear simulation has been carried out and two controllers are compared. Both optimal robust controllers guarantee robust performance of the system against the uncertainties and result in desired time responses of the output variables. By applying H ∞ robust control, system tracks the desire reference inputs in a less time and with smoother time responses.
Keywords: Energy consumption; Boiler pressure modelling; Model uncertainties; QFT; H∞Technique; µ-Synthesis; Genetic algorithm
1. Introduction1876-6102 © 2011 Published by Elsevier Ltd. Selection and/or peer-review under responsibility of the organizing committee of 2nd International Conference on Advances in Energy Engineering (ICAEE). doi:10.1016/j.egypro.2011.12.887
Available online at Available online at
Energy Procedia
/locate/procedia
Energy Procedia 00 (2011) 000–000 Energy Procedia 14 (2012) 1471 – 1477
© 2011 Published by Elsevier Ltd. Selection and/or peer-review under responsibility of the organizing committee © 2011 Published by Elsevier Ltd. Selection and/or peer-review under responsibility of [name organizer] of 2nd International Conference on Advances in Energy Engineering (ICAEE).
1472 2
S. Mostafavi et al.\ /Procedia Energy Procedia 14 (2012) 1471 – 1477 Mostafavi Toroghi et al. / Energy 00 (2011) 000–000
Boilers are commonly found in industry where they are used to supply turbines with steam or for heating of other chemical compounds. Although the steam production is varied during plant operation, output variables such as steam pressure must be maintained at their respected values. Therefore, tracking the load variation commands of drum pressure is expected from a power plant boiler system. However, the physical constraints exerted on the actuators must be satisfied by the control signals. These constraints can be the magnitude and saturation rate for the control valves of the fuel, steam and feed-water flow [1, 2]. A boiler unit is a nonlinear complex system. Several dynamic models of the boiler system have been developed [3]. Various control methods have been applied to boiler or boiler–turbine controller design, e.g., gain scheduling and feedback linearization [1, 2], quantitative Feedback Theory [4], and intelligent control [5-7]. Most practical systems have high uncertainty levels in their open-loop transfer functions which makes it very difficult to create suitable stability margins and good performance in command following problems for a closed-loop system. Therefore, a single fixed controller in such systems is found among the 'robust control' family. Some aspects related to robust control and mixed sensitivity [8, 9, 15] have been applied in different ways. Except in [9] in other works, the parametric uncertainties associated with dynamic model are not considered. A close loop system is robust if it is insensitive to differences between the actual system and the model of the system which was used to design the controller. These differences are referred to as model uncertainty. The H ∞ robust control technique is used to check if the design specifications are satisfied even for the worst-case uncertainty. In this approach, a mathematical representation of the model uncertainty is found. Then it is determined if the stability and performance specifications are satisfied for all plants in the uncertainty set (robust stability and robust performance) [10]. In this paper, a linear time invariant (LTI) model of the boiler system is considered which is obtained from experimental data. After modelling the parametric uncertainties, a QFT robust controller is designed. Then the uncertain system is represented in the form of general control configuration and unstructured uncertainty is considered in the form of multiplicative input uncertainty. On the other hand, to achieve the goals of disturbance rejection and command tracking, the sensitivity function must have a special shape which is obtained by considering a suitable performance weight function. Using an algorithm for µ-analysis and using genetic algorithm, an optimal robust controller is designed. Both optimal robust controller and QFT robust controller guarantee the robust performance of the uncertain system. The structure of the paper will be as follows. In section 2 the model for the plant is presented. Robust controller design procedure is presented in section 3. The QFT controller design will be presented in section 4 briefly, which is followed by an analysis of the robust stability and the robust performance in section 5. 2. Modeling 2.1 Physical system description