X-ray Emission from the Type Ic Supernova 1994I Observed with Chandra
The ASCA X-ray spectrum of the powerful radio galaxy 3C109
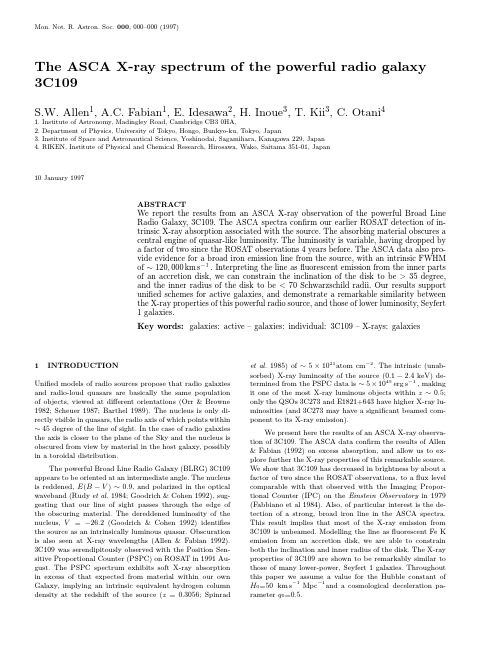
Mon.Not.R.Astron.Soc.000,000–000(1997)The ASCA X-ray spectrum of the powerful radio galaxy3C109S.W.Allen1,A.C.Fabian1,E.Idesawa2,H.Inoue3,T.Kii3,C.Otani41.Institute of Astronomy,Madingley Road,Cambridge CB30HA,2.Department of Physics,University of Tokyo,Hongo,Bunkyo-ku,Tokyo,Japan3.Institute of Space and Astronautical Science,Yoshinodai,Sagamihara,Kanagawa229,Japan4.RIKEN,Institute of Physical and Chemical Research,Hirosawa,Wako,Saitama351-01,Japan10January1997ABSTRACTWe report the results from an ASCA X-ray observation of the powerful Broad LineRadio Galaxy,3C109.The ASCA spectra confirm our earlier ROSAT detection of in-trinsic X-ray absorption associated with the source.The absorbing material obscures acentral engine of quasar-like luminosity.The luminosity is variable,having dropped bya factor of two since the ROSAT observations4years before.The ASCA data also pro-vide evidence for a broad iron emission line from the source,with an intrinsic FWHMof∼120,000km s−1.Interpreting the line asfluorescent emission from the inner partsof an accretion disk,we can constrain the inclination of the disk to be>35degree,and the inner radius of the disk to be<70Schwarzschild radii.Our results supportunified schemes for active galaxies,and demonstrate a remarkable similarity betweenthe X-ray properties of this powerful radio source,and those of lower luminosity,Seyfert1galaxies.Key words:galaxies:active–galaxies:individual:3C109–X-rays:galaxies1INTRODUCTIONUnified models of radio sources propose that radio galaxies and radio-loud quasars are basically the same population of objects,viewed at different orientations(Orr&Browne 1982;Scheuer1987;Barthel1989).The nucleus is only di-rectly visible in quasars,the radio axis of which points within ∼45degree of the line of sight.In the case of radio galaxies the axis is closer to the plane of the Sky and the nucleus is obscured from view by material in the host galaxy,possibly in a toroidal distribution.The powerful Broad Line Radio Galaxy(BLRG)3C109 appears to be oriented at an intermediate angle.The nucleus is reddened,E(B−V)∼0.9,and polarized in the optical waveband(Rudy et al.1984;Goodrich&Cohen1992),sug-gesting that our line of sight passes through the edge of the obscuring material.The dereddened luminosity of the nucleus,V=−26.2(Goodrich&Cohen1992)identifies the source as an intrinsically luminous quasar.Obscuration is also seen at X-ray wavelengths(Allen&Fabian1992). 3C109was serendipitously observed with the Position Sen-sitive Proportional Counter(PSPC)on ROSAT in1991Au-gust.The PSPC spectrum exhibits soft X-ray absorption in excess of that expected from material within our own Galaxy,implying an intrinsic equivalent hydrogen column density at the redshift of the source(z=0.3056;Spinrad et al.1985)of∼5×1021atom cm−2.The intrinsic(unab-sorbed)X-ray luminosity of the source(0.1−2.4keV)de-termined from the PSPC data is∼5×1045erg s−1,making it one of the most X-ray luminous objects within z∼0.5; only the QSOs3C273and E1821+643have higher X-ray lu-minosities(and3C273may have a significant beamed com-ponent to its X-ray emission).We present here the results of an ASCA X-ray observa-tion of3C109.The ASCA data confirm the results of Allen &Fabian(1992)on excess absorption,and allow us to ex-plore further the X-ray properties of this remarkable source. We show that3C109has decreased in brightness by about a factor of two since the ROSAT observations,to aflux level comparable with that observed with the Imaging Propor-tional Counter(IPC)on the Einstein Observatory in1979 (Fabbiano et al1984).Also,of particular interest is the de-tection of a strong,broad iron line in the ASCA spectra. This result implies that most of the X-ray emission from 3C109is unbeamed.Modelling the line asfluorescent Fe K emission from an accretion disk,we are able to constrain both the inclination and inner radius of the disk.The X-ray properties of3C109are shown to be remarkably similar to those of many lower-power,Seyfert1galaxies.Throughout this paper we assume a value for the Hubble constant of H0=50km s−1Mpc−1and a cosmological deceleration pa-rameter q0=0.5.22THE ASCA OBSER V ATIONSThe ASCA X-ray astronomy satellite(Tanaka,Inoue& Holt1994)consists of four separate nested-foil telescopes, each with a dedicated X-ray detector.The detectors in-clude two Solid-state Imaging Spectrometers or SISs(Burke et al.1991,Gendreau1995)and two Gas scintillation Imaging Spectrometers or GISs(Kohmura et al.1993). The SIS instruments provide high quantum efficiency and good spectral resolution,∆E/E=0.02(E/5.9keV)−0.5.The GIS detectors provide a lower resolution,∆E/E= 0.08(E/5.9keV)−0.5,but cover a larger(∼50arcmin diam-eter)circularfield of view.3C109was observed with ASCA on1995Aug28-29. The SIS observations were made in the standard1-CCD mode(Day et al.1995)with the source positioned at the nominal pointing position for this mode.X-ray event lists were constructed using the standard screening criteria and data reduction techniques discussed by Day et al.(1995). The observations are summarized in Table1.Source spectra were extracted from circular regions of radius4arcmin(SIS0),3.5arcmin(SIS1)and6arcmin (GIS2,GIS3),respectively.For the SIS data,background spectra were extracted from regions of the chip relatively free of source counts.For the GIS data,background spectra were extracted from circular regions,the same size as the source regions,and at similar distance from the optical axes of the telescopes.Spectral analysis was carried out using the XSPEC spectralfitting package(Shafer et al.1991).For the SIS data,the1994Nov9version of the SIS response matrices were used.For the GIS data the1995Mar6response ma-trices were used.The spectra were binned to have a mini-mum of20counts per Pulse Height Analysis(PHA)channel, thereby allowingχ2statistics to be used.In general,best-fit parameter values and confidence limits quoted in the text are the results from simultaneousfits to all4ASCA data sets,with the normalization of the power-law continuum al-lowed to vary independently for each data set.3RESULTS3.1Confirmation of excess X-ray absorptionin3C109The principal result of the ROSAT PSPC observation of 3C109(Allen&Fabian1992)was the detection of X-ray absorption in excess of the Galactic value determined from 21cm HI observations.The ASCA data allow us to verify and expand upon this result.The ASCA data werefirst examined using a simple ab-sorbed power law model.This allows direct comparison with the results of Allen&Fabian(1992).The free parameters in thefits were the column density of the absorbing mate-rial,N H,the photon index of the power law emission,Γ, (both parameters were forced to take the same value in all4 ASCA data sets)and the normalizations,A1,of the power-law emission.(Due to the range of source extraction regions used,and known systematic differences in theflux calibra-tion of the different ASCA detectors,the value of A1was allowed to vary independently for each data set).The best fit parameter values and90per cent(∆χ2=2.71)confi-Figure1.(Upper panel)The SIS and GIS spectra of3C109with the bestfitting absorbed power-law model(Model A)overlaid. (Lower panel)The residuals to thefit in units ofχ.(For plotting purposes the data have been rebinned along the energy axis by a factor7.)Figure2.(Upper panel)The ratio of data to model,where the model is the best-fit Model A,but with the absorption reset to the Galactic value(assumed to be3×1021atom cm−2).Note the large negative residuals at energies below2keV which are due to the excess absorption,and the evidence for a broad,redshifted emission line feature at∼5keV.For plotting purposes,the SIS (open circles)and GIS(filled squares)data sets have been av-eraged together and binned by a factor of20along the energy axis.The ASCA X-ray spectrum of the powerful radio galaxy 3C1093Table 1.Observation summaryInstrument Observation Date Exposure (ks)ASCA SIS01995Aug 28/2936.0ASCA SIS1””35.0ASCA GIS2””35.0ASCA GIS3””35.0ROSAT PSPC 1991Aug 3022.1Einstein IPC1979Mar 71.86Notes:X-ray observations of 3C109.Exposure times are for the final X-ray event lists after standard screening criteria and corrections have beenapplied.Figure 3.Joint confidence contours on the photon index and total column density,determined with spectral Model A (Table 3).Contours mark the regions of 68,90and 99per cent confidence (∆χ2=2.30,4.61and 9.21respectively).dence limits obtained with this simple model are presented in Table 3(Model A).The SIS and GIS spectra with their best-fitting models (Model A)overlaid are plotted in Fig.1.For illustrative purposes,in Fig.2we show the best fit model with the column density reset to the Galactic value (assumed to be 3.0×1021atom cm −2).Note the large negative residuals at energies,E <2keV,which demonstrate the effects of the excess absorption,and the broad positive residual at E ∼5keV,which will be discussed in more detail in Section 3.3.The ASCA results clearly confirm the PSPC result on excess absorption in the X-ray spectrum of 3C109.Assuming that the absorber lies at zero redshift we determine a total column density along the line of sight of 5.30±0.42×1021Figure 4.Joint confidence contours (68,90and 99per cent con-fidence)on the column density and redshift of the excess absorber in 3C109(using spectral Model B).atom cm −2(90per cent confidence limits).This is in goodagreement with the PSPC result of 4.2+1.9−1.6×1021atom cm −2.The ASCA result on the photon index,Γ=1.78+0.05−0.06,is also in excellent agreement with the PSPC result of1.78+0.85−0.76,although is more firmly constrained.The joint confidence contours on Γand N H are plotted in Fig.3.We have examined the constraints the ASCA spectra can place on the redshift of the excess absorbing mate-rial.The Galactic column density along the line of sight to 3C109,determined from 21cm observations,is 1.46×1021atom cm −2(Jahoda et al.1985;Stark et al.1992),although Johnstone et al.(1992)suggest a slightly higher value of ∼2.0×1021atom cm −2,and Allen &Fabian (1996)in-fer a value of ∼3.0×1021atom cm −2from X-ray stud-ies of the nearby cluster of galaxies Abell 478.Modelling4the ASCA spectra with a two-component absorber,with a Galactic(zero-redshift)column density of3.0×1021atom cm−2,and a component with variable column density and redshift,we obtain the joint confidence contours on the red-shift and column density of the excess absorption plotted in Fig.4.The best-fit parameter values and90per cent confidence limits for the two-component absorption model (Model B)are also summarized in Table3.3.2Variation of the X-ray luminosityTheflux measurements for3C109are summarized in Table2.Results are presented for both SIS instruments in the1.0−2.0and2.0−10.0keV(observer frame)energy bands.(The GIS detectors provide less accurateflux esti-mates).Also listed in Table2are thefluxes observed with the ROSAT PSPC in August1991and the IPC on Einstein Observatory in March1979.We see that in the overlapping 1.0−2.0keV energy band,the brightness of3C109has de-creased by a factor∼2since1991.Theflux determination from the ASCA data is now consistent with that inferred from the IPC observation in1979.Also listed in Table2are the intrinsic(absorption-corrected)X-ray luminosities of the source inferred from the observations.(Here the energy bands correspond to the rest-frame of the source).The absorption-corrected2−10keV luminosity inferred from the ASCA spectra is2.1×1045 erg s−1.(We assume that during the Einstein IPC observa-tions the source had the same spectral shape as determined from the ASCA observations.)3C109has also been observed to vary at near-infrared wavelengths.Rudy et al.(1984)found variations of a factor ∼2in the J band over afive year span from1978to1983. Elvis et al.(1984)similarly reported variations in the J,H and K bands of∼50per cent(in the same sense)on a timescale of2–3years between1980and1983.3.3Discovery of a broad iron lineThe residuals to thefits with the simple power-law mod-els,presented in Figs.1and2,exhibit an excess of counts in a line-like feature at E∼5.0keV.X-ray observations of Seyfert galaxies(Nandra&Pounds1994and references therein)show that many such sources exhibit a strong emis-sion line at E∼6.40keV(in the rest frame of the object). This is normally attributed tofluorescent Fe K emission from cold material irradiated by the nucleus.Wefind that thefit to the ASCA data for3C109is sig-nificantly improved by the introduction of a Gaussian line at E∼5keV(∆χ2=9.2for3extrafit parameters;an F-test indicates this to be significant at the97per cent level.)The best-fit line energy is5.09+0.44−0.38keV(corresponding to6.61+0.57−0.50keV in the rest frame of the source.Note that if afixed rest-energy of6.4keV is assumed,the introduction of the Gaussian component becomes significant at the∼99per cent confidence level).The data also indicate that the line isbroad,with a1sigma width of0.65+0.81−0.36keV.The equivalentwidth of the line is300+600−200eV.The width and energy of theline suggest that it is due tofluorescence from a rapidly ro-tating accretion disk–as is thought to be the case in lower luminosity Seyfert galaxies(Tanaka et al.1995;Fabianet Figure5.Joint confidence contours(68,and90per cent confi-dence)on the normalization,A2,and inclination,θ,of the disk line using spectral Model D(following Fabian et al.1989).al.1995).The bestfitting parameters and confidence limits for the power-law plus Gaussian model(Model C)are sum-marized in Table3.Note that the emission feature is not well-modelled by the introduction of an absorption edge at higher energies[the introduction of an edge into the simple absorbed power-law model(Model A)does not significantly improve thefit].Note also that the measured lineflux is not significantly affected by the small systematic bump in the XRT response at E∼5.5keV(which produces a nar-row,positive residual with aflux of a few per cent of the continuumflux at that energy).3.4Modelling the line as a disklineAlthough the simple Gaussian model provides a reason-able description of the5.0keV emission feature,the ASCA data suggest that the line profile is probably more ing two Gaussian components to model the line profile,we obtain the bestfit for a broad component with a rest energy consistent with6.4keV,and a narrow com-ponent with an energy6.8±0.1keV(in the rest-frame of the source).These results are similar to those obtained for nearby,lower-luminosity Seyfert galaxies(e.g.Mushotzky et al1995;Tanaka et al1995;Iwasawa et al.1996)where the line emission is thought to originate from the inner regions of an accretion disk surrounding a central,massive black hole(Fabian et al.1989).We have therefore modelled the broad line in3C109us-ing the Fabian et al.(1989)model for line emission from a relativistic accretion disk.The rest-energy of the line(in the emitted frame)wasfixed at6.40keV,the energy appropri-ate for Fe Kfluorescence from cold material.(The effects of cosmological redshift were incorporated into the model.) The accretion disk was assumed to extend over radii from3The ASCA X-ray spectrum of the powerful radio galaxy3C1095 Table2.X-rayflux of3C109Instrument Date F X L X2-10keV1-2keV2-10keV1-2keV ASCA SIS01995Aug28/2948.5±1.69.50±0.2321.4±0.36.69+0.76−0.71 ASCA SIS1””46.3±2.19.76±0.3221.3±0.37.68+1.10−0.890.1-2.4keV1-2keV0.1-2.4keV1-2keVROSAT PSPC1991Aug3028.9±2.618.2±0.745+147−2512.1+6.3−3.50.5-3.0keV1-2keV0.5-3.0keV1-2keVEinstein IPC1979Mar720±68.3±2.516.5±5.06.2±1.9Notes:The X-rayflux and luminosity of3C109measured with ASCA,ROSAT and the Einstein Observatory.Fluxes are in units of10−13erg cm−2s−1and are defined in the rest frame of the observer.Luminosities are in1044erg s−1,are absorption corrected,and are quoted in the rest frame of the source.Errors are90per cent(∆χ2=2.71)confidence limits.to500Schwarzschild radii(hereafter R s)and cover a solid angle of2πsteradians.The emissivity was assumed to follow a standard disk radiation law.Only the disk inclination and line strength were free parameters in thefit.The bestfit parameters and90per cent confidence limits obtained with the diskline model are listed in Table3(Model D).The in-troduction of the diskline component significantly improves thefit to the ASCA data with respect to the power-law model(∆χ2=8.9for2extrafit parameters,which an F-test indicates to be significant at the99per cent confidence level).In Fig.5we show the joint confidence contours on the inclination of the disk,θ,versus the line strength,A2.The 90per cent(∆χ2=2.71)constraint on the inclination is θ>35degree.We have also examined the constraints that may be placed on the inner radius,r in,of the accretion disk with the diskline model.The data were re-fitted with r in included as a free parameter.The preferred value for r in is3R s,with a90per cent confidence upper limit of70R s. (Note that for an ionized disk,with a rest-energy for the line of6.7keV,the inclination is constrained toθ>18degree.) The effects of introducing a further,flatter power-law component into thefits,such as may be required to ac-count for reflected emission from the illuminated face of an accretion disk,or synchrotron self-Compton emission from within a jet,were also examined.The introduction of aflat-ter power-law component does not significantly improve the fits.However,the ASCA spectra permit(with no significant change inχ2)the inclusion of a continuum spectrum appro-priate for reflection from a cold disk,subtending a solid angle of2πsteradians to the primary X-ray source,oriented at any inclination consistent with the results from the disklinefits. 4DISCUSSIONThe ASCA results on excess X-ray absorption in3C109con-firm and refine the earlier ROSAT results(Allen&Fabian 1992).The ASCA data show(under the assumption that all of the absorbing material lies at zero redshift)that theX-ray spectrum of the source is absorbed by a total col-umn density of5.30+0.42−0.42×1021atom cm−2(Model A).This compares to a Galactic column density of∼3.0×1021atom cm−2(Allen&Fabian1996).If we instead assume that the excess absorption,over and above the Galactic value,is due to material at the redshift of3C109,we determine an in-trinsic column density of4.20+0.83−0.78×1021atom cm−2.Note that these results assume solar abundances in the absorbing material(Morrison&McCammon1983).The X-ray absorption measurements are in good agree-ment with optical results on the polarization and intrinsic reddening of the source.Goodrich&Cohen(1992)deter-mine an intrinsic continuum reddening of E(B−V)∼0.9, in addition to an assumed Galactic reddening of E(B−V)= ing the standard(Galactic)relationship between E(B−V)and X-ray column density,N H/E(B−V)= 5.8×1021atom cm−2mag−1(Bohlin,Savage&Drake1978), the total reddening observed,E(B−V)∼1.2,implies a total X-ray column density(Galactic plus intrinsic)of∼7.0×1021 atom cm−2.This result is similar to the X-ray column den-sity inferred from the ASCA spectra using model B and confirms the presence of significant intrinsic absorption at the source.Note that this result also suggests that the dust-to-gas ratio in3C109is similar to that in our own Galaxy.Further constraints on the distribution of the absorbing gas are obtained from the optical emission-line data pre-sented by Goodrich&Cohen(1992).In the narrow line re-gion(NLR),the observed Blamer decrement of Hα/Hβ= 5.8implies(for an assumed recombination ratio of3.2)an E(B−V)value∼ing the relationship of Bohlin, Savage&Drake(1978)this implies an X-ray column den-sity to the NLR of∼2.8×1021atom cm−2,in good agree-ment with the Galactic column density of∼3.0×1021atom cm−2determined by Allen&Fabian(1996)and adopted in the X-ray analysis presented here.The Balmer decrement in the broad line region(BLR)is very steep(Hα/Hβ=13.2). Although this value cannot be reliably used to infer the ex-tinction to the BLR,the intrinsic line ratio is unlikely to6Table3.Results of the spectral analysisMODEL PARAMETERSAΓA1N H———χ2/DOFwabs(pow)1.78+0.05−0.061.38+0.10−0.100.530+0.042−0.042———638.9/633BΓA1N H N H(z)——χ2/DOFwabs zwabs(pow)1.77+0.05−0.061.35+0.10−0.090.3000.420+0.083−0.078——641.6/633CΓA1N H EσA2χ2/DOFwabs(pow+gau)1.86+0.12−0.081.47+0.16−0.120.558+0.056−0.0465.09+0.44−0.380.65+0.81−0.362.1+4.3−1.3629.7/630DΓA1N H EθA2χ2/DOFwabs(pow+diskline)1.87+0.08−0.081.48+0.14−0.130.561+0.052−0.0466.4090+0.0−552.4+1.4−1.4630.0/631Notes:A summary of best-fit parameters and90per cent(∆χ2=2.71)confidence limits from the spectral analysis of the ASCA data.Results are shown for four different modelsfitted simultaneously to the data for all four ASCA detectors.Γis the photon index of the underlying power-law continuum from the source.A1is the normalization of the power law component in the S0detector in10−3photon keV−1cm−2s−1at1keV.N H is the equivalent hydrogen column density in1022atom cm−2at zero redshift.In Model B,N H(z)is the best-fit intrinsic column density at the source for an assumed Galactic column density of0.3×1022atom cm−2.In Model C,E is the energy of the Gaussian emission line in the frame of the observer,σis the one-sigma line width in keV,and A2is the line strength in10−5photon cm−2s−1.In Model D,E is the rest-energy of the line in the emitted frame,θis the inclination of the disk in degree,and A2is again the line strength in10−5photon cm−2s−1.be above5,suggesting a total line-of sight reddening to the BLR of E(B−V)∼>0.8.Thus,the BLR is likely to be intrin-sically reddened by E(B−V)∼>0.3.The optical emission line results are therefore consistent with the two-component absorber model(B),with the column density of the intrinsic absorber being comparable with the Galactic component.3C109is the most powerful object in which a strong broad iron line has been resolved to date.Several more lu-minous quasars observed with ASCA do not show any iron emission or reflection features(Nandra et al1995).The next most luminous object with a confirmed broad line is 3C390.3(Eracleous,Halpern&Livio1996)which is about 10times less luminous in both the X-ray and radio bands than3C109.The equivalent widths of the lines in both ob-jects are∼300eV and therefore similar to those observed in lower-luminosity Seyferts.This argues against any X-ray ‘Baldwin effect’(as proposed by Iwasawa&Taniguchi1993).The line emission from3C109is most plausibly due to fluorescence from the innermost regions of an accretion disc around a central black hole(Fabian et al1995).Our results constrain the inner radius of the accretion disk to be<70R s and the inclination of the disk to be>35degree.The strong iron line observed in3C109,and the lack of evidence for a synchrotron self-Compton continuum in the X-ray spectrum, both suggest that little radiation from the jet is beamed into our line of sight.The inclination determined from the ASCA data is larger than the angle proposed by Giovannini et al.(1994) based on the jet/coreflux ratio of the source(θ<34de-gree).However,the jet/coreflux arguments are based on simple assumptions about the average orientation angles for radio galaxies and neglect environmental effects.The con-flict with the X-ray results may indicate that the situation is more complicated.Giovannini et al.(1994)also present constraints on the inclination from VLBI observations of the jet/counterjet ratio,which requireθ<56degree.The VLBI constraint,together with the ASCA X-ray constraint, then suggests35<θ<56degree.Our results on3C109are in good agreement with the unification schemes for radio sources and illustrate the power of X-ray observations for examining such models.The pre-ferred,intermediate inclination angle for the disk in3C109 is in good agreement with the results on X-ray absorption, polarization and optical reddening of the source,all of which suggest that our line of sight to the nucleus passes closes to the edge of the surrounding molecular torus.The results on the broad iron line reveal a striking similarity between the X-ray properties of3C109and those of lower power,Seyfert 1galaxies(Mushotzky et al1995;Tanaka et al1995;Iwa-sawa et al1996).This is despite the fact that the X-ray power of3C109exceeds that of a typical Seyfert galaxy by ∼2orders of magnitude.5ACKNOWLEDGEMENTSWe thank K.Iwasawa,C.Reynolds and R.Johnstone for discussions and the annonymous referee for helpful and con-structive comments concerning the intrinsic reddening in 3C109.SW A and ACF thank the Royal Society for support.The ASCA X-ray spectrum of the powerful radio galaxy3C1097 REFERENCESAllen S.W.&Fabian A.C.,1992,MNRAS,258,29PAllen S.W.&Fabian A.C.,1996,MNRAS,in pressBarthel P.D.,1989,ApJ,336,606Bohlin R.C.,Savage B.D.,Drake J.F.,1978,ApJ,224,132Burke B.E.et al.1991,IEEE Trans.,ED-38,1069Day C.,Arnaud K.,Ebisawa K.,Gotthelf E.,Ingham J.,MukaiK.,White N.,1995,the ABC Guide to ASCA DataReduction,NASA GSFCElvis M.,Willner S.P.,Fabbiano G.,Carleton N.P.,LawrenceA.,Ward M.,1984,ApJ,280,574Eracleous M.,Halpern J.P.,Livio M.,1996,ApJ,459,89Fabian A.C.,Rees M.J.,Stella L.,White N.E.,1989,MNRAS,238,729Fabian A.C.,Nandra K.,Reynolds C.S.,Brandt W.N.,OtaniC.,Tanaka Y.,1995,MNRAS,277,11LFabbiano G.,Miller L.,Trinchieri G.,Longair M.,Elvis M.,1984,ApJ,277,115Gendreau K.et al.,1995,PASJ,47,L5Giovanni G.et al.,1994,ApJ,435,116Goodrich R.W.,Cohen M.H.,1992,ApJ,391,623Iwasawa K.,Taniguchi Y.,1993,ApJ,413,15LIwasawa K.,Fabian A.C.,Mushotzky R.F.,Brandt W.N.,AwakiH.,Kunieda H.,1996,MNRAS,279,837Jahoda K.,McCammon D.,Dickey J.M.,Lockman F.J.,1985,ApJ,290,229Johnstone R.M.,Fabian A.C.,Edge A.C.,Thomas P.A.,1992,MNRAS,255,431Kohmura Y.et al.,1993,Proc.SPIE,2006,78Morrison R.,McCammon D.M.,1983,ApJ,270,119Mushotzky R.F.,Fabian A.C.,Iwasawa K.,Kunieda H.,Matsuoka M.,Nandra K.,Tanaka Y.,1995,MNRAS,272,9LNandra K.,Pounds K.A.,1994,MNRAS,268,405Nandra K.,et al.1995,MNRAS,276,1Orr M.J.L.,Browne I.W.A.,1982,MNRAS,200,1067Rudy R.J.,Schmidt G.D.,Stockman H.S.,Tokunaga,A.T.,1984,ApJ,278,530Scheuer P.A.G.,1987,in Zensus J.A.&Pearson T.E.,ed,Superluminal Radio Sources,Cambridge University Press,CambridgeShafer R.A.,Haberl F.,Arnaud K.A.,Tennant A.F.,1991,XSPEC User’s Guide,.ESA,NoordwijkSpinrad H.,Djorgovski S.,Marr J.,Aguilar L.,1985,PASP,97,932Stark A.A.,Gammie C.F.,Wilson R.W.,Bally J.,Linke R.A.,Heiles C.,Hurwitz M.,1992,ApJS,79,77Tanaka Y.,Inoue H.,Holt S.S.,1994,PASJ,46L,37Tanaka Y.et al.,1995,Nature,275,659。
x射线吸收谱xas的英文
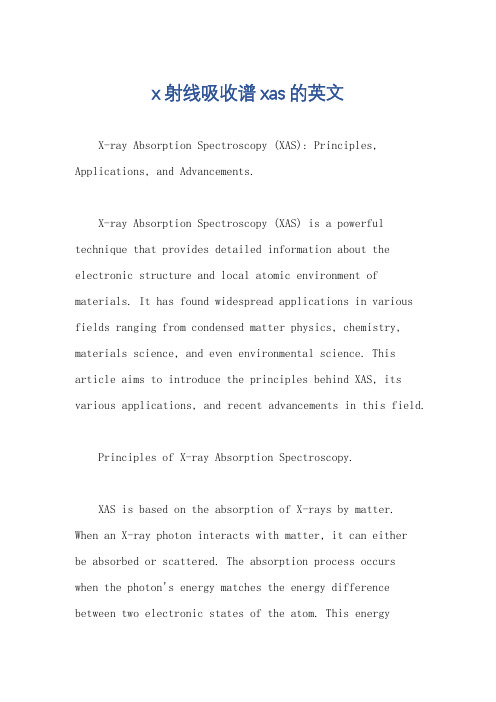
x射线吸收谱xas的英文X-ray Absorption Spectroscopy (XAS): Principles, Applications, and Advancements.X-ray Absorption Spectroscopy (XAS) is a powerful technique that provides detailed information about the electronic structure and local atomic environment of materials. It has found widespread applications in various fields ranging from condensed matter physics, chemistry, materials science, and even environmental science. This article aims to introduce the principles behind XAS, its various applications, and recent advancements in this field.Principles of X-ray Absorption Spectroscopy.XAS is based on the absorption of X-rays by matter. When an X-ray photon interacts with matter, it can eitherbe absorbed or scattered. The absorption process occurs when the photon's energy matches the energy difference between two electronic states of the atom. This energydifference is typically in the range of a few thousand to tens of thousands of electron volts, making X-rays an excellent probe for studying the inner electronic structure of atoms.In XAS experiments, a monochromatic X-ray beam is passed through a sample, and the intensity of the transmitted X-rays is measured as a function of energy. The absorption spectrum is then obtained by plotting the absorbed X-ray intensity against the incident X-ray energy. The shape and features of this spectrum are directlyrelated to the electronic structure and local atomic environment of the sample.There are two main types of XAS: X-ray Absorption Near-Edge Structure (XANES) and Extended X-ray Absorption Fine Structure (EXAFS). XANES probes the absorption near the absorption edge, providing information about the unoccupied states of the absorber atom. On the other hand, EXAFS focuses on the oscillatory structure that appears above the absorption edge and gives insights into the coordination geometry and bond lengths of the absorber atom.Applications of X-ray Absorption Spectroscopy.1. Materials Science: XAS is widely used in materials science to study the atomic-scale structure and electronic properties of various materials. It can provide information about the oxidation state, coordination geometry, and bond lengths of atoms in materials, which is crucial for understanding their physical and chemical properties.2. Chemistry: In the field of chemistry, XAS is often used to study the electronic structure and bonding in molecules and solids. It can reveal the oxidation states, ligand environments, and electronic configurations of atoms in complex molecules.3. Environmental Science: XAS has also found applications in environmental science, where it is used to study the speciation and transformation of elements in the environment. For example, it can be used to monitor the redox state of heavy metals in soils and waters, which is crucial for assessing their environmental risk.4. Energy Science: In the field of energy science, XAS is used to study materials for energy conversion and storage applications. For instance, it can provide insights into the electronic structure and atomic-scale structure of battery materials, which is essential for developing high-performance batteries.Advancements in X-ray Absorption Spectroscopy.With the continuous development of synchrotronradiation sources and advanced detector technologies, XAS has made significant advancements in recent years. Here are some of the key advancements in XAS:1. Higher Energy Resolution: Advancements in X-ray sources and detectors have led to improved energyresolution in XAS experiments. This higher resolution allows for more precise measurements of electronicstructure parameters and enhanced sensitivity to small changes in the local atomic environment.2. Faster Acquisition Times: With the advent of new detector technologies, XAS experiments can now be performed with much faster acquisition times. This reduction in measurement time not only improves experimental efficiency but also enables studies of dynamic processes and reactions in real time.3. Element-Specific Probing: Recent advancements in XAS have enabled element-specific probing, allowing researchers to study the electronic structure and local atomic environment of specific elements within a complex material. This capability is particularly useful in materials science and chemistry, where understanding the behavior of individual elements is crucial.4. In-Situ Studies: Another important advancement in XAS is the ability to perform in-situ studies, where the sample is exposed to various external stimuli (e.g., temperature, pressure, electric field) during the measurement. This approach allows researchers to study the evolution of materials' properties under realistic conditions, providing valuable insights into theirperformance and stability.In conclusion, X-ray Absorption Spectroscopy (XAS) is a powerful technique that provides detailed information about the electronic structure and local atomic environment of materials. Its applications span across various fields, and recent advancements in XAS have further expanded its capabilities and scope. With continued research and development, XAS is expected to play an even more critical role in understanding and developing advanced materials for various applications.。
X-ray Emission Diagnostics from the M87 Jet
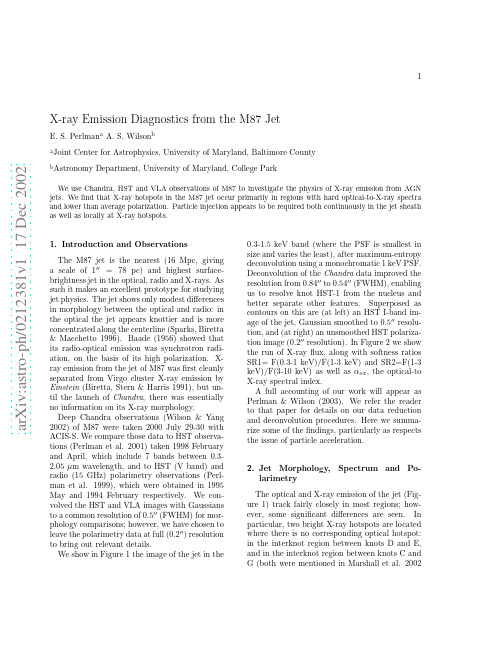
a r X i v :a s t r o -p h /0212381v 1 17 D e c 20021X-ray Emission Diagnostics from the M87JetE.S.Perlman a A.S.Wilson baJoint Center for Astrophysics,University of Maryland,Baltimore County bAstronomy Department,University of Maryland,College ParkWe use Chandra,HST and VLA observations of M87to investigate the physics of X-ray emission from AGN jets.We find that X-ray hotspots in the M87jet occur primarily in regions with hard optical-to-X-ray spectra and lower than average polarization.Particle injection appears to be required both continuously in the jet sheath as well as locally at X-ray hotspots.1.Introduction and ObservationsThe M87jet is the nearest (16Mpc,giving a scale of 1′′=78pc)and highest surface-brightness jet in the optical,radio and X-rays.As such it makes an excellent prototype for studying jet physics.The jet shows only modest differences in morphology between the optical and radio:in the optical the jet appears knottier and is more concentrated along the centerline (Sparks,Biretta &Macchetto 1996).Baade (1956)showed that its radio-optical emission was synchrotron radi-ation,on the basis of its high polarization.X-ray emission from the jet of M87was first cleanly separated from Virgo cluster X-ray emission by Einstein (Biretta,Stern &Harris 1991),but un-til the launch of Chandra ,there was essentially no information on its X-ray morphology.Deep Chandra observations (Wilson &Yang 2002)of M87were taken 2000July 29-30with ACIS-S.We compare those data to HST observa-tions (Perlman et al.2001)taken 1998February and April,which include 7bands between 0.3-2.05µm wavelength,and to HST (V band)and radio (15GHz)polarimetry observations (Perl-man et al.1999),which were obtained in 1995May and 1994February respectively.We con-volved the HST and VLA images with Gaussians to a common resolution of 0.5′′(FWHM)for mor-phology comparisons;however,we have chosen to leave the polarimetry data at full (0.2′′)resolution to bring out relevant details.We show in Figure 1the image of the jet in the0.3-1.5keV band (where the PSF is smallest in size and varies the least),after maximum-entropy deconvolution using a monochromatic 1keV PSF.Deconvolution of the Chandra data improved the resolution from 0.84′′to 0.54′′(FWHM),enabling us to resolve knot HST-1from the nucleus and better separate other features.Superposed as contours on this are (at left)an HST I-band im-age of the jet,Gaussian smoothed to 0.5′′resolu-tion,and (at right)an unsmoothed HST polariza-tion image (0.2′′resolution).In Figure 2we show the run of X-ray flux,along with softness ratios SR1=F(0.3-1keV)/F(1-3keV)and SR2=F(1-3keV)/F(3-10keV)as well as αox ,the optical-to X-ray spectral index.A full accounting of our work will appear as Perlman &Wilson (2003).We refer the reader to that paper for details on our data reduction and deconvolution procedures.Here we summa-rize some of the findings,particularly as respects the issue of particle acceleration.2.Jet Morphology,Spectrum and Po-larimetry The optical and X-ray emission of the jet (Fig-ure 1)track fairly closely in most regions;how-ever,some significant differences are seen.In particular,two bright X-ray hotspots are located where there is no corresponding optical hotspot:in the interknot region between knots D and E,and in the interknot region between knots C and G (both were mentioned in Marshall et al.200225101520220510152022Figure 1.Left panel.Deconvolved Chandra 0.3–1.5keV image of the jet with contours from a smoothed HST I-band (F814W)optical image.In both cases,the scaling is by the square root of image values.Right panel.Deconvolved Chandra 0.3–1.5keV image of the jet with contours from a full-resolution HST optical polarimetry image.The greyscaling is identical to the above;however,the contours begin at 5%polarization and go up to 60%.2002).In addition,the X-knots (E and F)are located upstream of their consistent with an X-ray 1.3.This has been re-components by Wilson et al.;however,our anal-knot HST-1(see also to fit a spectral in-There do,however,ap-in the softness ratios (Fig-significantly at distances The variations in SR1are increasing column in of the jet.Significant im-fits are obtained with .7×1020cm −2for the nu-by Wilson &Yang)and The variations in SR2,explained by an increasing N (H )values.Rather,of the jet spectrum at are too few photons in constrain this.more variation in αox HST-1has a much harder than any other knot,remainder of the inner jet steeper spectra at larger In addition to this small flattenings at the po-The additional flat-of localized particle accel-(see §3).The jet’s optical-to-X-ray spectrum becomes even steeper beyond knot A (12.4′′from the nucleus),reach-ing αox =1.8in knot C.Interestingly,the knot A-B-C complex does knot show significant spec-tral hardening near the knot maxima.X-ray flux is strongly anti-correlated with opti-cal polarization (Figure 1),although the details of the relationship differ in the inner and outer jet.In all the knots in the inner jet,the X-ray flux peak is located at a local polarization minimum with P <20%,similar to the optical flux-optical polarization anti-correlation noted in Perlman et al.(1999).Immediately upstream from inner3Figure 2.Top Panel.The run of X-ray flux in the deconvolved Chandra image.Plotted against this (with the same distance scale)are:Second from top.Optical-to-X-ray spectral index,Third and Fourth from the top.Softness ratios SR1and SR2,and Bottom.The comparison of predicted to observed 1keV flux from the continuous injec-tion synchrotron model.jet knot maxima we see increases in polarization and magnetic fields to the jet,while downstream from the knot maxima we see increased polariza-tion but magnetic fields parallel to the jet.In the outer jet,the anti-correlation between X-ray flux and optical polarization is weaker.Knot A’s maximum is located at a local minimum in po-larization although unlike the inner jet knots the polarization there is still appreciable (35%)rather than consistent with zero.The peak of knot B is also located in relatively low polarization regions,but there are also polarization minima in the knot A-B-C complex which do not correspond to X-ray maxima.In addition,the X-ray peak of knot C is located in a fairly high polarization region.3.Physical ImplicationsX-ray synchrotron emitting particles have life-times of only a few to tens of years assuming near-equipartition magnetic fields (e.g.,Meisenheimer,R¨o ser &Schl¨o telburg 1996,Heinz &Begelman 1997),meaning that in situ particle acceleration is required to produce the observed X-ray emis-sion extending over a jet 7000ly long.Can we identify loci of particle acceleration?In Figure 2one notices an excellent correlation (at least in the inner jet)between the loci of X-ray flux max-ima and the loci of flat optical-to-X-ray spectrum regions.Such spectral changes are suggestive of local particle acceleration in the knot maxima.However our modeling shows that to be insuffi-cient to explain the observed X-ray emission.We fit the radio to optical data with syn-chrotron spectrum models,and then use the mod-els to predict the X-ray flux and spectral index in each pixel.We have done this using the code of Leahy (1991)and Carilli et al.(1991).Three models were fit:(1)the Jaffe &Perola (1972)model,which assumes no continuous particle in-jection and but includes pitch-angle reisotropiza-tion;(2)the Kardashev (1962)and Pacholczyk (1970)model,which assumes neither particle in-jection nor pitch-angle scattering;and (3)a con-tinuous injection (CI:Heavens &Meisenheimer 1987)model,under which a power law distribu-tion of electrons is continuously injected.We show in the bottom panel of Figure 2the ratio4F pred/F obs at1keV for the continuous injection model.The Jaffe&Perola model is not shown because it underpredicts the X-rayflux by or-ders of magnitude at many places and predicts an exponential decay of the X-ray spectral index (not observed),while the Kardashev-Pacholczyk model is not shown because it consistently un-derpredicts the X-rayflux by large factors and predicts too steep a spectral index.Two main patterns can be seen in this plot. In most of the jet(except for knot HST-1), F pred/F obs∼1−10,meaning that particle accel-eration occurs within100-10%of the volume of the jet.There is a gradual increase in F pred/F obs as the distance from the nucleus increases.Small increases in F pred/F obs occur at knot maxima in the inner jet.This suggests that if particle accel-eration occurs within the knot maxima,as sug-gested by the optical-to-X-ray(this paper)and optical spectra(Perlman et al.2001)at these points,the loci of particle acceleration must be much smaller than Chandra can resolve.Knot HST-1appears to be a special case.It is the only place in the jet where the contin-uous injection model significantly underpredicts the X-rayflux.The reason for this is unclear. Knot HST-1is known to be very active,with superluminally moving components(Biretta et al.1999).Recently,HST-1has shown blazar-like X-ray’flaring’(Harris,these proceedings).It is possible that this variability plays a part in the anomalous F pred/F obs seen in HST-1.Al-ternately,an extra emission component could be present in the jet at higher energies;however,our modeling does not have sufficient angular resolu-tion to test this hypothesis.Importantly,we do not see large departures in the value of F pred/F obs in inter-knot regions. Moreover,the spectral models that do not include particle injection or acceleration still underpre-dict the X-ray emission at these loci by large fac-tors.Thus even in inter-knot regions wefind evi-dence of continuous particle injection,most likely operating in the sheath of the M87jet.A similar conclusion was reached by Jester et al.(2001)for 3C273on the basis of radio-optical data. Interestingly,we see different polarization sig-natures in the regions where our modeling indi-cates in situ particle acceleration.As shown in Figure1and Perlman et al.(1999),the sheath of the M87jet exhibits high polarization and mag-neticfields parallel to the jet,while the knot max-ima have either low or no polarization.Thus while the knots appear to be shock-like features (Perlman et al.1999),where Fermi acceleration may be the dominant process,a different mecha-nism may operate in the sheath. REFERENCES[1]Baade,W.,1956,ApJ,123,550[2]Biretta,J.A.,Stern,C.P.,&Harris,D.E.,1991,AJ,101,1632[3]Carilli,C.L.,Perley,R.A.,Dreher,J.W.,&Leahy,J.P.,1991,ApJ,383,554[4]Harris,D.E.,these proceedings[5]Heavens,A.,&Meisenheimer,K.,1987,MN-RAS,225,335[6]Heinz,S.,&Begelman,M.C.,1997,ApJ,490,633[7]Jaffe,W.J.,&Perola,G.C.,1973,A&A,26,421[8]Jester,S.,R¨o ser,H.–J.,Meisenheimer,K.,Perley,R.,Conway,R.,2001,A&A,373,447 [9]Kardashev,N.S.,1962,Soviet AstronomyAJ,6,317[10]Leahy,J.P.,1991,in Beams and Jets in As-trophysics(Cambridge),p.100][11]Meisenheimer,K.,R¨o ser,H.-J.,&Shl¨o telburg,M.,1996,A&A,307,61] [12]Perlman, E.S.,Biretta,J. A.,Zhou, F.,Sparks,W.B.,Macchetto,F.D.,1999,AJ, 117,2185[13]Perlman,E.S.,Biretta,J.A.,Sparks,W.B.,Macchetto,F.D.,Leahy,J.P.,2001,ApJ, 551,206[14]Sparks,W.B.,Biretta,J.A.,&Macchetto,F.D.,1996,ApJ,473,254[15]Wilson,A.S.,&Yang,Y.,2002,ApJ,568,133。
x射线荧光光谱法 英文
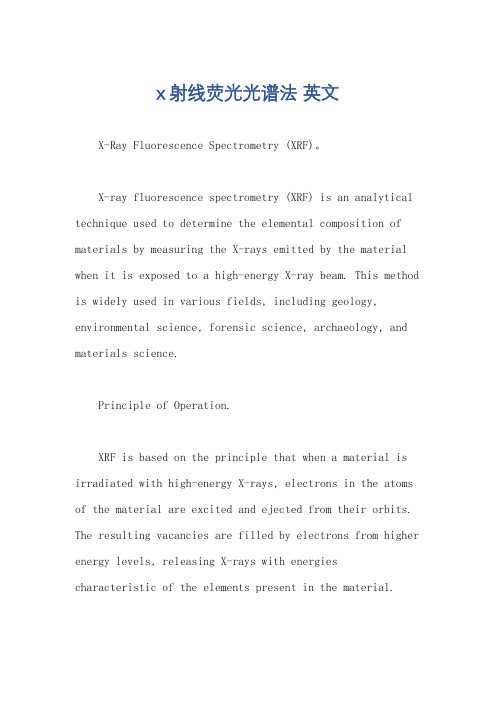
x射线荧光光谱法英文X-Ray Fluorescence Spectrometry (XRF)。
X-ray fluorescence spectrometry (XRF) is an analytical technique used to determine the elemental composition of materials by measuring the X-rays emitted by the material when it is exposed to a high-energy X-ray beam. This method is widely used in various fields, including geology, environmental science, forensic science, archaeology, and materials science.Principle of Operation.XRF is based on the principle that when a material is irradiated with high-energy X-rays, electrons in the atoms of the material are excited and ejected from their orbits. The resulting vacancies are filled by electrons from higher energy levels, releasing X-rays with energiescharacteristic of the elements present in the material.The energy of the emitted X-rays is specific to each element, and the intensity of the X-rays is proportional to the concentration of the element in the material. By measuring the energies and intensities of the emitted X-rays, it is possible to identify and quantify the elements present in the sample.Instrumentation.A typical XRF spectrometer consists of the following components:X-ray source: Generates high-energy X-rays that bombard the sample.Sample chamber: Holds the sample to be analyzed.Detector: Converts X-rays into electrical signals.Multichannel analyzer (MCA): Digitizes and analyzes the electrical signals from the detector.Types of XRF Spectrometers.There are several types of XRF spectrometers, each with its own advantages and limitations:Energy-dispersive XRF (EDXRF): Uses a solid-state detector to measure the energies of the emitted X-rays. EDXRF is relatively inexpensive and easy to operate, but it has lower energy resolution compared to other types of XRF spectrometers.Wavelength-dispersive XRF (WDXRF): Uses a crystal monochromator to separate the emitted X-rays by wavelength. WDXRF offers higher energy resolution than EDXRF, but it is more complex, expensive, and time-consuming to operate.Total reflection XRF (TXRF): Utilizes total reflection conditions to enhance the sensitivity for analyzing trace elements in liquids. TXRF is highly sensitive, but it requires sample preparation and is not suitable for solid samples.Applications of XRF.XRF is a versatile analytical technique with a wide range of applications:Geochemistry: Determining the elemental composition of rocks, minerals, and soils.Environmental science: Monitoring pollutants in air, water, and soil.Forensic science: Analyzing trace evidence, such as gunshot residue and paint chips.Archaeology: Studying the composition of artifacts and ancient materials.Materials science: Characterizing the elemental composition of metals, alloys, and other materials.Advantages of XRF.Nondestructive: Does not damage the sample being analyzed.Multi-elemental: Can identify and quantify multiple elements simultaneously.Rapid: Provides real-time analysis results.Sensitive: Can detect elements at trace levels.Versatile: Can be applied to various sample types, including solids, liquids, and powders.Limitations of XRF.Limited sensitivity: Cannot detect elements present in very low concentrations.Matrix effects: The presence of other elements in the sample can affect the accuracy of the analysis.Sample preparation: May require sample preparation,such as grinding or homogenization.Cost: XRF spectrometers can be expensive, especially WDXRF systems.Conclusion.X-Ray Fluorescence Spectrometry is a powerful analytical technique that provides valuable information about the elemental composition of materials. It is widely used in various fields and offers advantages such as non-destructiveness, multi-elemental analysis, and rapid results. However, it has limitations in sensitivity and potential matrix effects, which should be considered when selecting this technique for specific applications.。
天文学名词英汉对照表
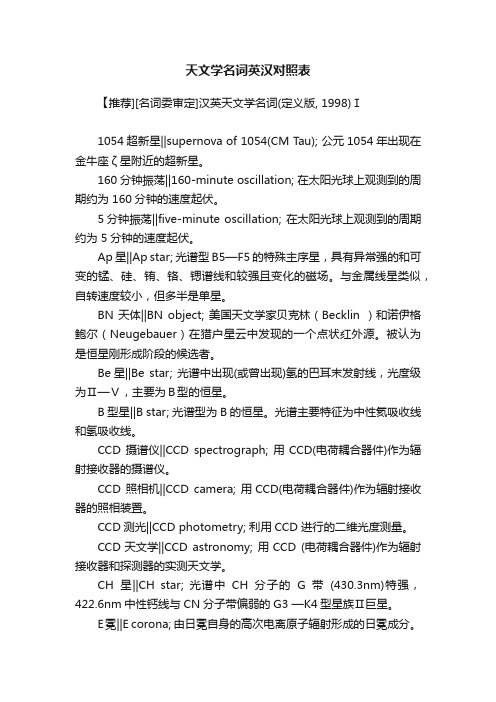
天文学名词英汉对照表【推荐][名词委审定]汉英天文学名词(定义版, 1998)Ⅰ1054超新星||supernova of 1054(CM T au); 公元1054年出现在金牛座ζ星附近的超新星。
160分钟振荡||160-minute oscillation; 在太阳光球上观测到的周期约为 160分钟的速度起伏。
5分钟振荡||five-minute oscillation; 在太阳光球上观测到的周期约为 5分钟的速度起伏。
Ap星||Ap star; 光谱型B5—F5的特殊主序星,具有异常强的和可变的锰、硅、铕、铬、锶谱线和较强且变化的磁场。
与金属线星类似,自转速度较小,但多半是单星。
BN天体||BN object; 美国天文学家贝克林(Becklin )和诺伊格鲍尔(Neugebauer)在猎户星云中发现的一个点状红外源。
被认为是恒星刚形成阶段的候选者。
Be星||Be star; 光谱中出现(或曾出现)氢的巴耳末发射线,光度级为Ⅱ—Ⅴ,主要为B型的恒星。
B型星||B star; 光谱型为B的恒星。
光谱主要特征为中性氦吸收线和氢吸收线。
CCD 摄谱仪||CCD spectrograph; 用CCD(电荷耦合器件)作为辐射接收器的摄谱仪。
CCD 照相机||CCD camera; 用CCD(电荷耦合器件)作为辐射接收器的照相装置。
CCD测光||CCD photometry; 利用CCD 进行的二维光度测量。
CCD天文学||CCD astronomy; 用CCD (电荷耦合器件)作为辐射接收器和探测器的实测天文学。
CH星||CH star; 光谱中CH分子的G带(430.3nm)特强,422.6nm中性钙线与CN分子带偏弱的G3 —K4型星族Ⅱ巨星。
E冕||E corona; 由日冕自身的高次电离原子辐射形成的日冕成分。
F 冕||F corona; 由行星际尘埃云散射太阳光球辐射形成的日冕成分。
G型星||G star; 光谱型为G的恒星。
Diffuse X-Ray Emission from the Quiescent Superbubble M17, the Omega Nebula
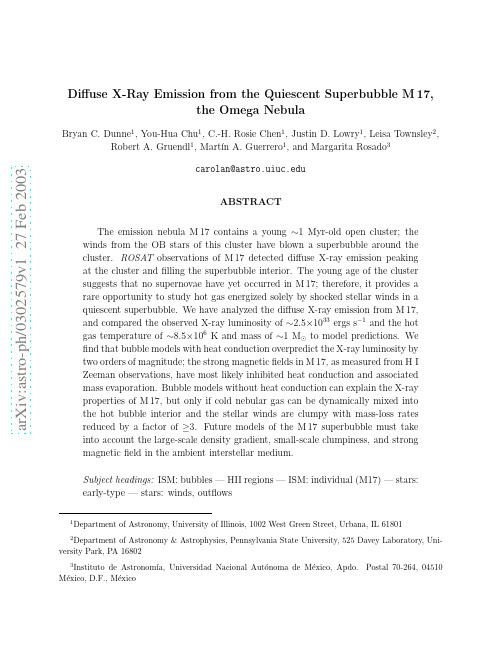
a rXiv:as tr o-ph/32579v127Fe b23Diffuse X-Ray Emission from the Quiescent Superbubble M 17,the Omega Nebula Bryan C.Dunne 1,You-Hua Chu 1,C.-H.Rosie Chen 1,Justin D.Lowry 1,Leisa Townsley 2,Robert A.Gruendl 1,Mart´ın A.Guerrero 1,and Margarita Rosado 3carolan@ ABSTRACT The emission nebula M 17contains a young ∼1Myr-old open cluster;the winds from the OB stars of this cluster have blown a superbubble around the cluster.ROSAT observations of M 17detected diffuse X-ray emission peaking at the cluster and filling the superbubble interior.The young age of the cluster suggests that no supernovae have yet occurred in M 17;therefore,it provides a rare opportunity to study hot gas energized solely by shocked stellar winds in a quiescent superbubble.We have analyzed the diffuse X-ray emission from M 17,and compared the observed X-ray luminosity of ∼2.5×1033ergs s −1and the hot gas temperature of ∼8.5×106K and mass of ∼1M ⊙to model predictions.We find that bubble models with heat conduction overpredict the X-ray luminosity by two orders of magnitude;the strong magnetic fields in M 17,as measured from H I Zeeman observations,have most likely inhibited heat conduction and associated mass evaporation.Bubble models without heat conduction can explain the X-ray properties of M 17,but only if cold nebular gas can be dynamically mixed into the hot bubble interior and the stellar winds are clumpy with mass-loss rates reduced by a factor of ≥3.Future models of the M 17superbubble must takeinto account the large-scale density gradient,small-scale clumpiness,and strong magnetic field in the ambient interstellar medium.Subject headings:ISM:bubbles —HII regions —ISM:individual (M17)—stars:early-type —stars:winds,outflows1.IntroductionMassive stars dynamically interact with the ambient interstellar medium(ISM)via their fast stellar winds and supernova ejecta.OB associations,with their large concentrations of massive stars,provide an excellent laboratory to study these interactions.The combined actions of the stellar winds and the supernovae from the massive stars in OB associations sweep up the ambient ISM to form expanding shells called superbubbles(Bruhweiler et al. 1980).The physical structure of a superbubble is very similar to that of a bubble blown by the stellar wind of an isolated massive star,as modeled by Castor,McCray,&Weaver (1975)and Weaver et al.(1977).Theoretically,an interstellar bubble consists of a shell of swept-up ISM with its interior filled by shocked fast wind at temperatures of106–108K.There are two basic types of models for wind-blown bubbles:energy-conserving and momentum-conserving.In the former,the shocked stellar wind is separated from the swept-up interstellar shell by a contact discontinu-ity,where heat conduction and mass evaporation may take place.The expansion of the shell is driven by the pressure of the hot interior gas(Dyson&de Vries1972;Castor et al.1975). In the momentum conserving bubbles,the fast stellar winds impinge on the swept-up shell directly,and the expansion is driven by the momentum of the fast stellar wind(Avedisova 1972;Steigman,Strittmatter,&Williams1975).One significant difference between a superbubble and a single star bubble is the possibil-ity that supernovae may occur inside a superbubble and introduce significant perturbations in the surface brightness and characteristic temperature of the X-ray emission,especially if a supernova explodes near the dense shell(Mac Low&McCray1988).This intermittent X-ray brightening has been observed in superbubbles in the Large Magellanic Cloud(LMC). Using Einstein and ROSAT observations,Chu&Mac Low(1990)and Dunne,Points,& Chu(2001)have reported diffuse X-ray emission from a large number of superbubbles in the LMC,and their X-ray luminosities all exceed the luminosities expected by Weaver et al.’s bubble model,indicating recent heating by supernovae.No LMC superbubbles in a quies-cent state,i.e.,without recent supernova heating,have been detected in X-rays by ROSAT (Chu et al.1995).It would be of great interest to detect diffuse X-ray emission from a qui-escent superbubble and compare it to model expectations,as this could provide a valuable diagnostic of bubble models.The emission nebula M17(from the catalog of Messier1850,α=18h21m,δ=−16◦10′(J2000.0),also known as the Omega Nebula,the Horseshoe Nebula,and NGC6618)is located on the eastern edge of a massive molecular cloud,M17SW(Lada,Dickinson,&Penfield 1974).M17exhibits a“blister-like”structure with an overall diameter of∼20′–25′,or∼10–12pc at an adopted distance of1.6kpc(Nielbock et al.2001).The nebula encompassesan open cluster with a stellar age of∼1Myr(Hanson,Howarth,&Conti1997).The open cluster is located on the western side of the nebula,which borders the molecular cloud. Arcuatefilaments extend eastward,creating a shell morphology,and suggesting that it is a young superbubble blown by the OB stars within.The young age of the cluster in M17 implies that no supernova explosions have occurred,thus M17provides an ideal setting to study the generation of hot gas solely by fast stellar winds inside a superbubble at a quiescent state.Recent Chandra observations of M17have revealed diffuse X-ray emission in the vicinity of the embedded open cluster(Townsley et al.2003).As a matter of fact,diffuse X-ray emis-sion from M17over a more extended area was previously detected in a ROSAT observation but was never reported.We have analyzed this diffuse X-ray emission from the interior of M17detected by ROSAT to determine the physical properties of the hot interior gas,and considered bubble models with and without heat conduction.Wefind that models with heat conduction produce results with the largest discrepancy from the observed X-ray luminos-ity and hot gas temperature and mass of the superbubble in M17.This paper reports our analysis of the ROSAT observations of M17and comparisons to a range of bubble models.2.Observations and Data Reduction2.1.ROSAT Archival DataWe have used an archival ROSAT Position Sensitive Proportional Counter(PSPC) observation to study the diffuse X-ray emission from M17and investigate the physical prop-erties of the hot,shocked gas interior to the superbubble.The PSPC is sensitive to X-rays in the energy range0.1–2.4keV and has an energy resolution of∼40%at1keV,with a field of view of∼2◦.Further information on the PSPC can be found in the ROSAT Mission Description(1991).The PSPC observation of M17(sequence number RP500311,PI:Aschenbach)was ob-tained on1993September12–13.It is centered on the nebula atα(J2000)=18h21m04.s78 andδ(J2000)=−16◦10′12.′′0,and has an exposure time of6.7ks.As M17has an angular size of∼20′–25′,the diffuse X-ray emission from the superbubble interior is well contained within the inner window support ring of the PSPC.The PSPC data were reduced using standard routines in the PROS4package under the IRAF5environment.2.2.Optical ImagingTo compare the spatial distribution of the X-ray-emitting gas with that of the cooler ionized gas in M17,we have obtained narrow-band Hαimages of this emission nebula.M17 was observed with the Mount Laguna1-m telescope on2002October28–31using a Tektronik 2K CCD.Afilter with peak transmission at6563˚A and a FWHM of20˚A was used to isolate the Hαline.Because the2K CCD has∼0.′′4pixels and afield-of-view of13.′6,the entire nebula could not be observed in a single exposure.Instead a total of twenty-four300s exposures were acquired at8positions to span the nebula.These exposures were combined to form a mosaic image of the nebula and to reject cosmic-ray events in the individual images using the methods outlined in Regan&Gruendl(1995).3.Analysis of the X-Ray EmissionSignificant X-ray emission is detected from M17in the PSPC observation,as can be seen in Figure1.To determine the nature and origin of this emission,we have analyzed its spatial distribution and spectral properties.We have examined the distribution of the X-ray emission and compared it to the optical morphology of the emission nebula.We have also extracted spectra from the PSPC data and modeled them to determine the physical conditions of the hot gas.3.1.Spatial Distribution of the X-ray EmissionTo study the spatial distribution of the X-ray emission from M17,the data were binned to5′′pixels and then smoothed with a Gaussian function ofσ=4pixels(see Figure1a). We have also taken our Hαmosaic and overlaid it with X-ray emission contours to study the extent of X-ray emission within the H II region(see Figure1b).Diffuse X-ray emission is observed to be well confined by the optical nebula.This diffuse emission shows no evidence of limb brightening,suggesting that the interior of M17is centrallyfilled with hot gas. The peak of the X-ray emission is coincident with the center of the open cluster in M17, where Chandra observations show a large number of point sources superposed on the diffuse emission(Townsley et al.2003).This spatial distribution suggests that the X-ray-emitting hot gas originates in the open cluster,as would be expected in a bubble blown by stellarwinds.As there is a massive molecular cloud to the west of M17,we expect the hot gas to expand more rapidly to the east;thisflow of hot gas to the east has then blown the blister-like bubble seen in optical images.Four additional point sources are detected in thefield around M17.The brightest of these point sources lies to the south of the X-ray peak and has been previously designated 1WGA J1820.6−1615in the WGA Catalog of ROSAT Point Sources(White,Giommi,& Angelini1994).This point source is coincident with OI352(Ogura&Ishida1976),an O8star on the southern edge of the open cluster in M17.The other three point sources lie on the northern edge of the emission nebula.These X-ray point sources,designated as1WGA J1820.8−1603,1WGA J1821.0−1600,and1WGA J1820.8−1556,are coincident with stars GSC606265−01977,SAO161369(a known O5star),and GSC06265−01808, respectively.These point sources are marked in Figure1a.3.2.X-Ray SpectraIn order to examine the spectra of the diffuse X-ray emission,wefirst excluded the point sources found in§3.1.Then,noting that M17is on the edge of a dense molecular cloud,we have sectioned the nebula into four regions to account for anticipated changes in the foreground absorption column density.These regions have been labeled A,B,C,and D and are displayed in Figure2.Additionally,we have selected a background annulus around the superbubble,as indicated in Figure2.The background-subtracted spectra were then extracted from the PSPC eventfiles.The observed X-ray spectra of the superbubble is a convolution of several factors:the intrinsic X-ray spectrum of the superbubble,the intervening interstellar absorption,and the PSPC response function.Because the interstellar absorption and the PSPC response function are dependent on photon energy,we must assume models of the intrinsic X-ray spectrum and the interstellar absorption to make the problem tractable.As the X-ray emission from the superbubble interior appears largely diffuse,we have used the Raymond &Smith(1977)thermal plasma emission model to describe the intrinsic X-ray spectra of the superbubble and the Morrison&McCammon(1983)effective absorption cross-section per hydrogen atom for the foreground absorption,assuming solar abundances for both the emitting and absorbing materials.We then simulated the observed spectrum,combining theassumed models for the intrinsic spectrum and the interstellar absorption with the responsefunction of the PSPC.The best-fit spectrum is found by varying parameters and comparingχ2for the simulated and observed spectra.We performed aχ2grid search of simulated spectralfits to determine the best-fit levelsfor the thermal plasma temperature,kT,and absorption column density,N H.Plots of thebestfits to the X-ray spectra are shown in Figure3,andχ2plots are presented in Figure4.Theχ2plots of regions A,B,and C indicate that the X-ray emission can befit by eitherhigher temperature plasma,∼0.7keV,with lower absorption column density,∼1020−21cm−2,or lower temperature plasma,∼0.2keV,with higher absorption column density,∼1022cm−2.This is a common problem for PSPC spectra with a limited number of counts because ofthe poor spectral resolution and soft energy coverage of the PSPC.The best modelfits favorthe higher temperature plasma and lower absorption column density solution.Indeed,thedetection of soft X-ray emission below0.5keV indicates that the solution with N H∼1022cm−2cannot be valid,as such a solution predicts no significant soft X-ray emission should bedetected.Furthermore,the high absorption column density solution predicts a foregroundabsorption column density for M17equal to the total Galactic H I column density along theline of sight(Dickey&Lockman1990).As M17is located in the plane of the Galaxy atl=15◦03′,b=−00◦40′and has a distance of1.6kpc,we do not expect the majority of theGalactic H I toward this direction to be located in front of the superbubble.From the modelfits,we calculated the unabsorbed X-rayflux,and therefore the X-rayluminosity,L X,of the diffuse X-ray emission from each source region.The normalizationfactor,A,of the thermal plasma model is equal to n e n p dV/4πD2,where n e and n p are the electron and proton number densities,V is the volume of the superbubble,and D is thedistance to the source.Assuming a He:H number ratio of1:10and that the X-ray emittinggas is completely ionized,wefind n e≃1.2n p,and that the volume emission measure can beexpressed as<n2e>fV,where f is the volumefilling factor.We have used the diameter ofthe superbubble,∼10–12pc,as the depth of the X-ray emitting gas in each source region.We determined the volume,V,of each source region by multiplying the surface area of theregion by the depth and a geometric correction factor of2/3(approximated by the volumeratio of a sphere to a cylinder).Taking the volumefilling factor to be f=0.5,we thencalculated rms n e in each region.The best-fit values of kT,A,N H,L X and rms n e are givenin Table1.Note that the modelfit to region D did not converge because it contains a largenumber of unresolved stellar sources as well as diffuse emission;only approximate values forthe X-ray luminosity and absorption column density are given.See Townsley et al.(2003)for a detailed analysis of the Chandra observations of region D.Combining our results from each of the source regions,wefind a total diffuse X-ray lu-minosity of∼2.5×1033ergs s−1in the ROSAT PSPC0.1–2.4keV band,a mean characteristic temperature of kT∼0.72keV or T∼8.5×106K,a mean electron density of∼0.09cm−3,and a total hot gas mass of∼1M⊙.We have calculated the total thermal energy in hot,shocked wind component of the superbubble to be E th∼1×1048ergs with a cooling timescale of t T∼40Myr.Given the stellar age of the open cluster,∼1Myr,we do not expect significant radiative cooling to have occurred.As a rough check,we multiply the current X-ray lumi-nosity by the age of the cluster andfind that the total energy radiated away by the X-ray emission is 10%of the total thermal energy.4.Discussionparisons with Model ExpectationsWe now compare the observed physical properties of M17determined above to theo-retical calculations from basic wind-blown bubble models.Although the M17superbubble is in an inhomogeneous ambient medium with a significant density gradient and the cluster is off-centered(a more complex scenario than is considered in basic bubble models),if the superbubble structure is indeed governed by the physical processes prescribed by these mod-els,we expect the properties of the diffuse X-ray emission to agree with predictions within similar orders of magnitude.Wefirst consider the wind-blown bubble model of Weaver et al.(1977)and will later consider a wind-blown bubble model without heat conduction.4.1.1.A Bubble with Heat ConductionIn the Weaver et al.model,heat conduction and mass evaporation act across the bound-ary between the hot interior gas and the nebular shell to lower the temperature and raise the density of the bubble interior.The temperature and electron density profiles of such a bubble have been calculated by Weaver et al.(1977),and the X-ray luminosities of such bubbles can be determined using two methods outlined by Chu et al.(1995).In thefirst method,we derive the expected X-ray luminosity from the observed physical properties of the gas in the104K ionized shell of swept-up ISM.In the second method,we use the spectral types of massive stars in M17to estimate the combined mechanical luminosity of the stellar winds and then derive the expected X-ray luminosity.These two methods use independent input parameters and thus allow us to check the consistency of the pressure-driven bubble model in addition to comparing the expected and observed X-ray luminosities.X-Ray Luminosity Method1:The Ionized ShellThe expected X-ray luminosity in the ROSAT band of0.1–2.4keV for the Weaver et al. (1977)wind-blown bubble model has been given by Chu et al.(1995),L X=(8.2×1027ergs s−1)ξI(τ)n10/70R17/7pcV16/7km/s,(1)whereξis the metallicity relative to the solar value and in this case we assume a value of unity,I(τ)is a dimensionless integral of value∼2,n0is the number density of the ambient medium in cm−3,R pc is the radius of the superbubble in pc,V km/s is the expansion velocity of the superbubble in units of km s−1.The ambient density n0cannot be measured directly, but assuming that the ram pressure of the expanding shell is equal to the thermal pressure of the ionized superbubble shell,the relation between the ambient density and the density of the ionized shell is given byn0=(9/7)n i kT i/(µa V2exp),(2) where n i is the electron number density in the ionized shell,T i∼104K is the electron temperature in the ionized shell,V exp is the expansion velocity of the bubble,andµa= (14/11)m H(Weaver et al.1977;Chu&Mac Low1990).Adopting a mean electron density of n i∼300cm−3(Felli,Churchwell,&Massi1984)and an observed V exp∼25km s−1(Clayton et al.1985),we calculated an ambient density of n0∼40cm−3.Given the superbubble radius of5–6pc,we have determined an expected X-ray luminosity of∼3×1035ergs s−1.X-Ray Luminosity Method2:Wind Luminosity from OB StarsWe can also calculate the expected X-ray luminosity in an energy-conserving,wind-blown bubble by the following equation from Chu et al.(1995),L X=(1.1×1035ergs s−1)ξI(τ)L33/3537n17/35t19/35Myr,(3)where L37is the mechanical luminosity of the stellar winds in units of1037ergs s−1,and t Myr is the age of the bubble in Myr.To remain independent of Method1,we do not use the value of n0determined for that method.Rather,we use the following relations between ambient density,radius,wind luminosity,bubble age,and expansion velocity,n0=(1.3×108cm−3)L37t3Myr R−5pc,(4)t Myr=(0.59Myr)R pc/V km/s,(5) (Weaver et al.1977;Chu et al.1995).We again take the expansion velocity to be∼25km s−1 (Clayton et al.1985)and the radius to be5–6pc and derive a bubble age of∼0.13Myr.To determine the wind luminosity of M17,we examined its massive stellar content. Hanson et al.(1997)identified nine O stars and four late-O/early-B stars in the open cluster. Using the spectral types of these massive stars,we have estimated their terminal stellar wind velocities,effective temperatures,and luminosities based on the stellar parameters given by Prinja,Barlow,&Howarth(1990)and Vacca,Garmany,&Shull(1996).We then calculated the mass-loss rates for the OB stars in M17by utilizing the empirically derived relationship between effective temperature,luminosity,and mass-loss rate of de Jager,Nieuwenhuijzen,& van der Hucht(1988).Table2lists the detected OB stars,their spectral types as determined from optical and K-band observations,their terminal wind velocities V∞,stellar effective temperatures T eff,stellar luminosities L,and their mass-loss rates˙M.We calculated the total mechanical luminosity of the stellar winds,L w=Σ(1/2)˙MV2∞,(6) from the OB stars to be∼1×1037ergs s−1.As noted by Felli et al.(1984),the identified OB stars can approximately account for the ionization of the emission nebula;we therefore expect our calculated wind mechanical luminosity to be reasonably complete as well.Assuming a relatively constant mechanical luminosity,wefind a total energy deposited by stellar winds of∼4×1049ergs over the life of the bubble.In addition,this value of the wind mechanical luminosity gives an ambient density of∼60cm−3and an expected X-ray luminosity of ∼5×1035ergs s−1.This X-ray luminosity value is consistent to within a factor of two with the value found by Method1.Although the two methods of determining the expected X-ray luminosity are consistent with each other,they do not agree with the X-ray luminosity derived from the PSPC obser-vation.The observed X-ray luminosity is∼100–200times lower than expected from Weaver et al.’s bubble model.It is possible that stellar winds are clumpy,as suggested by Moffat& Robert(1994),then the conventionally derived mass loss rates would be reduced by a factor of≥3.Even using the reduced mass loss rates,the expected X-ray luminosity is more than 40times too high.The observed temperature,density,and surface brightness of the hot gas in M17do not agree with the model expectations,either.The physical conditions of the shocked stellar winds in Weaver et al.’s model are heavily modified by heat conduction and the hot gas mass is dominated by the nebular mass evaporated across the interface.The predicted temperature is5.0–5.6×106K near the center and decreases outward,the predicted density is0.2–0.4cm−3near the center and increases outward,and the X-ray surface bright-ness is expected to show pared with observed properties,the expected temperature is too low,density is too high,and the X-ray morphology is inconsistent.The disagreements between observations and model expectations suggest that heat conduction may not play a dominant role in determining the physical conditions inside this superbubble.Heat conduction can be suppressed by the presence of magneticfields(Soker1994;Band &Liang1988).The magneticfield strength in M17has been measured via the H I Zeeman effect to be100–550µG,peaking near the interface between the H II region and the molecular cloud M17SW(Brogan et al.1999).Assuming a comparable magneticfield strength in the swept-up104K shell,wefind the Alfv´e n speed to be10–60km s−1which is comparable to or much greater than the isothermal sound velocity of the104K gas,10km s−1for H atoms. In addition,the magneticfield strength and isothermal sound velocity indicate a gyro-radius of 10km for protons in the swept-up shell.This suggests that the protons in the104K gas will be unable to escape the magneticfield and diffuse into the interior of the superbubble, inhibiting heat conduction and mass evaporation between the hot interior and the cool shell of the bubble.4.1.2.A Bubble without Heat ConductionWe now turn our consideration to a wind-blown bubble without heat conduction.The X-ray emission of a bubble interior depends on both the temperature and the amount of hot gas.We willfirst compare the plasma temperature expected from the shocked stellar winds to the observed hot gas ing the combined stellar winds mass-loss rate of 4.3×10−6M⊙yr−1(summed from Table2)and the integrated wind mechanical luminosity L w of1×1037ergs s−1as calculated in§4.1.1,we derive an rms terminal wind velocity of V∞∼2700km s−1.The post-shock temperature of the combined stellar winds is therefore expected to be∼8×107K.This temperature is an order of magnitude higher than that indicated by PSPC observations;to lower it to the observed temperature of∼8.6×106K requires the mixing in of cold nebular mass that is nearly10times the mass of the combined stellar winds.This mixing may be provided by turbulent instabilities at the interface between the shocked fast winds and the cold nebular shell(e.g.,Strickland&Stevens1998)or through the hydronamic ablation of clumps of cold nebular material distributed within the hot bubble interior(Pittard,Hartquist,&Dyson2001).Assuming that mixing has taken place,we next determine the hot gas mass expected as a result of mixing and compare it to the observed value(§3.2).Given the dynamical age of the superbubble,0.13Myr,a total stellar wind mass of∼0.56M⊙has been injected to the superbubble interior,and the expected total mass of the hot gas will be5–6M⊙.This is significantly greater than the observed value of∼1M⊙.This discrepancy can be reduced if we again consider the possibility of clumpy stellar winds(Moffat&Robert1994).With the mass-loss rates reduced by a factor of≥3,the expected hot gas mass is∼2M⊙,which would be in remarkable agreement with the observed value.We summarize the comparison between the observed X-ray emission and the various models in Table3,which lists the observed X-ray luminosity and hot gas temperature and mass as well as those expected from models with and without heat conduction for both homogeneous winds and clumpy winds.It is clear that bubble models with heat conduction have the largest discrepancies from the observations.The best agreement with observed properties is from bubble models without heat conduction but allowing dynamical mixing of cold nebular material with the hot gas.For models either with or without heat conduction, clumpy winds with reduced mass loss rates are needed to minimize the discrepancy between model expectations and observations.parisons with Other Wind-Blown BubblesDiffuse X-ray emission has been previously detected from other types of wind-blown bubbles,including planetary nebulae(PNe)and circumstellar bubbles blown by Wolf-Rayet (WR)stars.The X-ray emission from these circumstellar bubbles is qualitatively and quan-titatively different from that of M17.Chu,Gruendl,&Guerrero(2003)find that the X-ray emission from PNe and WR bubbles shows a limb-brightened spatial distribution,in sharp contrast to the centrally-filled spatial distribution in M17as described in§3.1.Further,Chu et al.(2003)note that PNe and WR bubbles exhibit hot gas temperatures of1–3×106K and electron densities of10–100cm−3,while the hot interior gas of M17exhibits a temperature of8.5×106K and a substantially lower electron density of∼0.09cm−3.The comparisons of morphology and temperature between the M17superbubble and small circumstellar bubbles show fundamental differences.The limb-brightened X-ray spa-tial distribution,low temperatures,and high electron densities of PNe and WR bubbles are qualitatively consistent with a hypothesis of heat conduction and mass evaporation occurring between the hot gas interior and the swept-up shell.However,the observed X-ray luminosi-ties for PNe and WR bubbles are both significantly lower(10–100times)than predicted by bubble models with heat conduction(Chu et al.2001;Wrigge,Wendker,&Wisotzki1994; Wrigge1999).It is possible that in these wind-blown bubbles,heat conduction has also been suppressed and that dynamical mixing,which allows a lower mass injection rate,occurs at the interface between the hot gas interior and the cool nebular shell.Exploring this question will require magneticfield measurements of PNe and WR bubbles.5.SummaryWe have presented analysis of a ROSAT observation of the emission nebula M17.The blister-like morphology seen in the optical images indicates that it is a superbubble blown by the winds of its OB stars in an inhomogeneous ISM.With a stellar age of∼1Myr,M17must be a young quiescent superbubble without any supernova heating.Diffuse X-ray emission is detected from M17and is confined within the optical shell.This suggests the presence of hot106–108K gas in the interior of M17,as is expected in a wind-blown bubble.Analysis of the diffuse X-ray emission indicates a characteristic gas temperature∼8.5×106K with a mean electron number density of0.09cm−3.We have considered bubble models with and without heat conduction and found that those with heat conduction overpredict the X-ray luminosity by two orders of magnitude. Furthermore,the magneticfield measured in M17is large enough to suppress heat conduc-tion and associated mass evaporation.Bubble models without heat conduction overestimate the hot gas temperature unless mixing with cold nebular gas has occurred.If nebular gas can be dynamically mixed into the hot bubble interior and if the stellar winds are clumpy with a lower mass-loss rate,the X-ray morphology and luminosity,and hot gas temperature and mass can be reasonably reproduced.M17provides us a rare opportunity to probe the physical conditions of hot gas energized solely by shocked stellar winds in a quiescent superbubble.While we have learned much from the current analysis,our model considerations were performed on a very basic level.More robust models are needed to accurately describe the evolution of a superbubble in a medium with a large-scale density gradient,small-scale clumpiness,and a strong magneticfield.We would like to thank the anonymous referee for the stimulating comments with have helped us to improve this paper.This research has made use of data obtained through the High Energy Astrophysics Science Archive Research Center Online Service,provided by the NASA/Goddard Space Flight Center.。
TO APPEAR IN APJ LETTERS Preprint typeset using LATEX style emulateapj v. 101003 QUIESCENT
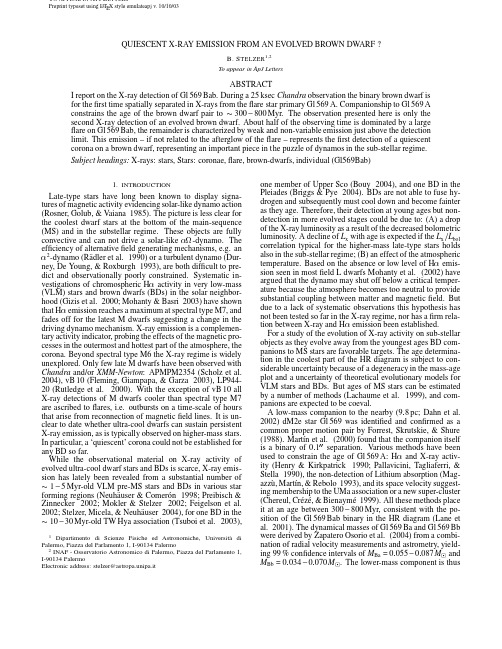
T O APPEAR IN A P J L ETTERSPreprint typeset using L A T E X style emulateapj v.10/10/03QUIESCENT X-RAY EMISSION FROM AN EVOLVED BROWN DW ARF?B.S TELZER1,2To appear in ApJ LettersABSTRACTI report on the X-ray detection of Gl569Bab.During a25ksec Chandra observation the binary brown dwarf isfor thefirst time spatially separated in X-rays from theflare star primary panionship to Gl569A constrains the age of the brown dwarf pair to300−800Myr.The observation presented here is only the second X-ray detection of an evolved brown dwarf.About half of the observing time is dominated by a large flare on Gl569Bab,the remainder is characterized by weak and non-variable emission just above the detection limit.This emission–if not related to the afterglow of theflare–represents thefirst detection of a quiescent corona on a brown dwarf,representing an important piece in the puzzle of dynamos in the sub-stellar regime.Subject headings:X-rays:stars,Stars:coronae,flare,brown-dwarfs,individual(Gl569Bab)1.INTRODUCTIONLate-type stars have long been known to display signa-tures of magnetic activity evidencing solar-like dynamo action (Rosner,Golub,&Vaiana1985).The picture is less clear for the coolest dwarf stars at the bottom of the main-sequence (MS)and in the substellar regime.These objects are fully convective and can not drive a solar-like-dynamo.The efficiency of alternativefield generating mechanisms,e.g.an 2-dynamo(Rädler et al.1990)or a turbulent dynamo(Dur-ney,De Young,&Roxburgh1993),are both difficult to pre-dict and observationally poorly constrained.Systematic in-vestigations of chromospheric H activity in very low-mass (VLM)stars and brown dwarfs(BDs)in the solar neighbor-hood(Gizis et al.2000;Mohanty&Basri2003)have shown that H emission reaches a maximum at spectral type M7,and fades off for the latest M dwarfs suggesting a change in the driving dynamo mechanism.X-ray emission is a complemen-tary activity indicator,probing the effects of the magnetic pro-cesses in the outermost and hottest part of the atmosphere,the corona.Beyond spectral type M6the X-ray regime is widely unexplored.Only few late M dwarfs have been observed with Chandra and/or XMM-Newton:APMPM2354(Scholz et al. 2004),vB10(Fleming,Giampapa,&Garza2003),LP944-20(Rutledge et al.2000).With the exception of vB10all X-ray detections of M dwarfs cooler than spectral type M7 are ascribed toflares,i.e.outbursts on a time-scale of hours that arise from reconnection of magneticfield lines.It is un-clear to date whether ultra-cool dwarfs can sustain persistent X-ray emission,as is typically observed on higher-mass stars. In particular,a‘quiescent’corona could not be established for any BD so far.While the observational material on X-ray activity of evolved ultra-cool dwarf stars and BDs is scarce,X-ray emis-sion has lately been revealed from a substantial number of 1−5Myr-old VLM pre-MS stars and BDs in various star forming regions(Neuhäuser&Comerón1998;Preibisch& Zinnecker2002;Mokler&Stelzer2002;Feigelson et al. 2002;Stelzer,Micela,&Neuhäuser2004),for one BD in the 10−30Myr-old TW Hya association(Tsuboi et al.2003), 1Dipartimento di Scienze Fisiche ed Astronomiche,Universitàdi Palermo,Piazza del Parlamento1,I-90134Palermo2INAF-Osservatorio Astronomico di Palermo,Piazza del Parlamento1, I-90134PalermoElectronic address:stelzer@astropa.unipa.it one member of Upper Sco(Bouy2004),and one BD in the Pleiades(Briggs&Pye2004).BDs are not able to fuse hy-drogen and subsequently must cool down and become fainter as they age.Therefore,their detection at young ages but non-detection in more evolved stages could be due to:(A)a drop of the X-ray luminosity as a result of the decreased bolometric luminosity.A decline of L x with age is expected if the L x L bol correlation typical for the higher-mass late-type stars holds also in the sub-stellar regime;(B)an effect of the atmospheric temperature.Based on the absence or low level of H emis-sion seen in mostfield L dwarfs Mohanty et al.(2002)have argued that the dynamo may shut off below a critical temper-ature because the atmosphere becomes too neutral to provide substantial coupling between matter and magneticfield.But due to a lack of systematic observations this hypothesis has not been tested so far in the X-ray regime,nor has afirm rela-tion between X-ray and H emission been established.For a study of the evolution of X-ray activity on sub-stellar objects as they evolve away from the youngest ages BD com-panions to MS stars are favorable targets.The age determina-tion in the coolest part of the HR diagram is subject to con-siderable uncertainty because of a degeneracy in the mass-age plot and a uncertainty of theoretical evolutionary models for VLM stars and BDs.But ages of MS stars can be estimated by a number of methods(Lachaume et al.1999),and com-panions are expected to be coeval.A low-mass companion to the nearby(98pc;Dahn et al. 2002)dM2e star Gl569was identified and confirmed as a common proper motion pair by Forrest,Skrutskie,&Shure (1988).Martín et al.(2000)found that the companion itself is a binary of01separation.Various methods have been used to constrain the age of Gl569A:H and X-ray activ-ity(Henry&Kirkpatrick1990;Pallavicini,Tagliaferri,& Stella1990),the non-detection of Lithium absorption(Mag-azzù,Martín,&Rebolo1993),and its space velocity suggest-ing membership to the UMa association or a new super-cluster (Chereul,Crézé,&Bienaymé1999).All these methods place it at an age between300−800Myr,consistent with the po-sition of the Gl569Bab binary in the HR diagram(Lane et al.2001).The dynamical masses of Gl569Ba and Gl569Bb were derived by Zapatero Osorio et al.(2004)from a combi-nation of radial velocity measurements and astrometry,yield-ing99%confidence intervals of M Ba=0055−0087M and M Bb=0034−0070M.The lower-mass component is thus2Stelzer thefirst object confirmed as substellar independent of models.In this Letter I present the Chandra detection of Gl569Bab, including a largeflare and probably thefirst detection of qui-escent X-ray emission from an evolved BD.2.OBSERV ATIONSThe Gl569system was observed with the Advanced CCD Imaging Spectrometer(ACIS),using the S3chip in imaging mode for a total exposure time of25ks.The data analysis was carried out using the CIAO software package3version2.3in combination with the calibration database(CALDB)version2.21.I started the analysis with the level1eventsfile pro-vided by the pipeline processing at the Chandra X-ray Center (CXC).In the process of converting the level1eventsfile toa level2eventsfile for each of the observations the following steps were performed:Charge transfer inefficiency(CTI)cor-rection,removal of the pixel randomization,filtering for event grades(retaining the standard ASCA grades0,2,3,4,and6),and application of the standard good time interval(GTI)file.The eventsfile was also checked for any systematic aspect offsets using CIAO software,but none were present.3.DATA ANALYSIS AND RESULTSThe expected position of Gl569A was found by translatingits Hipparcos position to the time of the Chandra observationwith help of the proper motion given by King et al.(2003).The position of Gl568Bab relative to the primary was ob-tained using the separation and P.A.of Lane et al.(2001). Source detection was carried out with the wavdetect algorithmon an image of5050pixels length(1pixel=0492)cen-tered on the computed position of the primary using wavelet scales between1and8in steps ofX-rays from Gl 569Bab30500010000150002000025000Time elapsed [s]0510********C o u n t sBin = 400 sGl569 B500010000150002000025000Time elapsed [s]102030405060C o u n t sBin = 400 sGl569 A F IG .2.—Source and background lightcurves of Gl 569Bab and Gl 569A.T ABLE 1X-RAY LUMINOSITY AND L x L bol RATIO FOR G L 569B AB .log L x [erg/s]258275268log (L x L bol )Ba−44−27−34log (L x L bol )Bb −42−25−324Stelzer56789log Age [yr]l o g (L x /L b o l ) [e r g /s ]F IG .5.—Ratio of L x L bol versus age for BDs:triangles,diamonds,open circles –bona-fide BDs in star forming regions (spectral types M7)with a random one order of magnitude spread in age centered on 05Myr for Oph (X-ray data from I01),15Myr for IC 348(PZ02),and 2Myr for Cha I (St04);flaring BDs are displayed as an asterisk,and those with accretion signatures in theH profile as x-points.References for evolved BDs:DENIS J1556(B04),TWA-5B (T03),2MW-J1207(GB04),Roque 14and four undetected BDs in the Pleiades (BP04),LP944-20(R00),and Gl 569Bab (this paper).the quiescent coronal activity for ultra-cool dwarfs conjec-tured by Fleming,Giampapa,&Garza (2003)must then be questioned.As seen from the upper limits in Fig.4,indeed,most previous observations of late M dwarfs were not sen-sitive enough to sample the range expected from an extrap-olation of the L x L bol values of the M0...M6dwarfs.How-ever,the non-detection of any photon from Gl 569Bab in the 22ks before the flare casts some doubt on this view.It isobserved occasionally that stars do not go back into their pre-flare quiescent state immediately after an outburst,thus we may see some kind of ‘afterglow’of the flare rather than true quiescent emission.On the other hand,based on the post-flare emission level a total of 26source photons are expected prior to the flare,consistent with the observation of zero photons at the 2limit.The detection of a strong X-ray flare on a comparatively evolved BD demonstrates that coronae of VLM objects can remain powerful beyond the youngest ages.Fig.5puts the Chandra observation of Gl 569Bab in context with observa-tions of younger BDs,and the only previous X-ray detection of a field BD,LP 944-20.A factor of 4−5more photons have been collected for Gl 569Bab with respect to all other late M dwarfs,allowing for the first time a meaningful spec-tral analysis of such an object.The spectrum shows signifi-cant emission above 1keV ,but a similarly strong cool com-ponent of 32MK.Low coronal temperatures seem to be characteristic for all but the youngest BDs.This is all the more remarkable as temperatures are known to rise in stellar flares.The flare temperatures of the evolved BDs (Gl 569Bab and LP 944-20)are similar to the quiescent temperature of the middle-aged BD TW A-5B on the one hand and to the quies-cent temperature of the evolved star vB 10on the other hand.Upcoming observations with Chandra sampling the age and temperature space shall constrain the influence of these pa-rameters on coronal emission.I would like to thank G.Micela and E.Flaccomio for fruit-ful discussions and careful reading of the manuscript.REFERENCESBouy,H.2004,A&A,424,619(B04)Briggs,K.R.,&Pye,J.P.2004,MNRAS,353,673(BP04)Chereul,E.,Crézé,M.,&Bienaymé,O.1999,A&AS,135,5Dahn,C.C.,Harris,H.C.,Vrba,F.J.,et al.2002,AJ,124,1170Durney,B.R.,De Young,D.S.,&Roxburgh,I.W.1993,Sol.Phys.,145,207Feigelson,E.D.,Broos,P.,Gaffney,J.A.III,et al.2002,ApJ,574,258Fleming,T.A.,Giampapa,M.S.,Schmitt,J.H.M.M.,&Bookbinder,J.A.1993,ApJ,410,387(F93)Fleming,T.A.,Schmitt,J.H.M.M.,&Giampapa,M.S.1995,ApJ,450,401(F95)Fleming,T.A.,Giampapa,M.S.,&Schmitt,J.H.M.M.2000,ApJ,533,372(F00)Fleming,T.A.,Giampapa M.S.,&Garza,D.2003,ApJ,594,982(F03)Forrest,W.J.,Skrutskie,M.F.,&Shure,M.1988,ApJ,330,L119Gizis,J.E.,&Bharat,R.2004,ApJ,608,L113(GB04)Gizis,J.E.,Monet,D.G.,Reid,I.N.,et al.2000,AJ,120,1085Gorlova,N.I.,Meyer,M.R.,Rieke,G.H.,&Liebert,J.2003,ApJ,593,1074Hambaryan V .,Staude,A.,Schwope,A.D.,et al.2004,A&A,415,265(H04)Hawley,S.L.,Gizis,J.E.,&Reid,I.N.1996,AJ,112,2799Henry,T.J.,&Kirkpatrick,J.D.1990,ApJ,354,L29Hünsch,M.,Schmitt,J.H.M.M.,Sterzik,M.F.,&V oges,W.1999,A&AS,135,319Imanishi,K.,Tsujimoto,M.,&Koyama,K.2001,ApJ,563,361(I01)King J.R.,Villarreal,A.R.,Soderblom,D.R.,Gulliver,A.F.,&Adelman,S.J.2003,AJ,125,1980Lachaume,R.,Dominik,C.,Lanz,T.,&Habing,H.J.1999,A&A,348,897Lane B.F.,Zapatero Osorio,M.R.,Britton,M.C.,Martin,E.L.,&Kulkarni,S.R.ApJ,560,390Magazzù,A.,Martín,E.L.,&Rebolo,R.1993,ApJ,404,L17Martín,E.L.,Koresko,C.D.,Kulkarni,S.R.,et al.2000,ApJ,529,L37Martín,E.L.,&Bouy,H.2002,New Astron,7,595Mohanty,S.,&Basri,G.2003,ApJ,583,451Mohanty,S.,Basri,G.,Shu,F.,Allard,F.,&Chabrier,G.2002,ApJ,571,469Mokler,F.,&Stelzer,B.2002,A&A,391,1025Neuhäuser,R.,&Comerón,F.1998,Science,282,83Neuhäuser,R.,Briceño,C.,Comerón,F.,et al.1999,A&A,343,883(N99)Pallavicini,R.,Tagliaferri,G.&Stella,L.1990,A&A,228,403Preibisch,T.,&Zinnecker,H.2002,AJ,123,1613(PZ02)Rädler,K.-H.,Wiedemann,E.,Meinel,R.,et al.1990,A&A,239,413Rosner,R.,Golub,L.,&Vaiana,G.S.1985,ARA&A,23,413Rutledge,R.E.,Basri,G.,Martín,E.L.,&Bildsten,L.2000,ApJ,538,L141(R00)Schmitt,J.H.M.M.,&Liefke,C.2002,A&A,382,L9(SL02)Scholz,R.-D.,Lodieu,N.,Ibata,R.,et al.2004,MNRAS ,347,685(S04)Stelzer,B.,Micela,G.,&Neuhäuser,R.2004,A&A,in press (St04)Tsuboi,Y .,Maeda,Y .,Feigelson,E.D.,et al.2003,ApJ,587,L51(T03)Zapatero Osorio,M.R.,Lane,B.L.,Pavlenko,Y .,et al.2004,ApJ,in press。
A Cluster of Galaxies hiding behind M31 XMM-Newton observations of RX J0046.4+4204

a r X i v :a s t r o -p h /0307223v 2 21 D e c 2005The Astrophysical Journal,acceptedPreprint typeset using L A T E X style emulateapj v.11/26/04A CLUSTER OF GALAXIES HIDING BEHIND M31:XMM-NEWTON OBSERVATIONS OF RXJ0046.4+4204Oleg Kotov 1,2,3,Sergey Trudolyubov 1,2,4,and W.Thomas Vestrand 1The Astrophysical Journal,acceptedABSTRACTWe report on our serendipitous discovery with the XMM-Newton Observatory of a luminous X-ray emitting cluster of galaxies that is located behind the Andromeda galaxy (M31).X-ray emission from the cluster was detected previously by ROSAT,and cataloged as RX J0046.4+4204,but it was not recognized as a galaxy cluster.The much greater sensitivity of our XMM-Newton observations revealed diffuse x-ray emission that extends at least 5′and has a surface brightness profile that is well fit by the α-βmodel with β=0.70±0.08,a core radius r c =56′′±16′′,and α=1.54±0.25.A joint global spectral fit of the EPIC/MOS1,MOS2,and PN observations with Mewe-Kaastra-Liedahl plasma emission model gives a cluster temperature of 5.5±0.5keV.The observed spectra also show high significance iron emission lines that yield a measured cluster redshift of z =0.290with a 2%accuracy.For a cosmological model with H 0=71km s −1Mpc −1,ΩM =0.3and ΩΛ=0.7we derive a bolometric luminosity of L x =(8.4±0.5)×1044erg/s.This discovery of a cluster behind M31demonstrates the utility of x-ray surveys for finding rich clusters of galaxies,even in directions of heavy optical extinction.Subject headings:galaxies:clusters:Intergalactic medium -X-rays:observation -Cosmology1.INTRODUCTIONGalaxy clusters are the largest gravitationally bound structures in the universe.The evolution of cluster num-ber density of a given mass is sensitive to specific cos-mological scenarios (e.g.Press &Schechter (1974)).So observations of galaxy clusters are an important tool for constraining fundamental cosmological parameters.Due to the fact that 15%of the total cluster mass (e.g.Evrard (1997))is in the form of hot diffuse plasma emit-ting at X-ray band via thermal bremsstrahlung (Sarazin 1988),galaxy clusters are among the most luminous ob-jects in X-ray band.It makes X-ray selection an efficient means for constructing samples of galaxy clusters (see review by Rosati et al.(2002)).X-ray selection has the advantage that the measurable X-ray luminosity and temperature are correlated with the cluster mass.Further,X-ray selection is useful for studying regions where optical searches are complicated because of dust extinction and heavy stellar confusion.X-rays are much less affected by extinction than optical photons and X-ray selection is almost free from source confusion prob-lems (Ebeling et al.2002).Conducting X-ray selection based on ROSAT data at low Galactic latitude,Ebeling et al.(2002)were able to detect 137galaxy clusters ,70%of which were new discoveries.With a new era of XMM-Newton and Chandra ob-servatories with their large effective areas and wide en-ergy ranges 0.3−10keV,the capability of X-ray selec-tion increased.During an XMM-Newton observation of the galactic supernova remnant G21.5-09located close to the Galactic Plane,Nevalainen et al.(2001)detected1NIS-2,Space and Remote Sciences Group,Los Alamos Na-tional Laboratory,Los Alamos,NM 875452Space Research Institute,Russian Academy of Sciences,Moscow,Russia3Harvard-Smithsonian Center for Astrophysics,60Garden St.,Cambridge,MA 021384Institute of Geophysics and Planetary Physics,University of California,Riverside,CA 92521a new galaxy ing only XMM-Newton data,they measured cluster redshift z =0.1to 1%precision that is especially important in regions with such strong optical source confusion,where the optical redshift mea-surements of galaxies are difficult .Here we present new XMM-Newton observations of the source RX J0046.4+4202that indicate it is a high red-shift cluster located behind M31.RX J0046.4+4204was detected during the first and the second deep ROSAT RSPC surveys of M31performed in June 1991and July/August 1992respectively (Supper et al.2001).Based on a comparison of the first and the second sur-veys,RX J0046.4+4204was classified as a potentially long term variable source.Our analysis of the data ob-tained with XMM-Newton revealed spatially extended emission,up to at least 5′,from RX J0046.4+4204.The observed spectra show iron emission lines that yield a measured redshift of z =0.290with a 2%accuracy.All these facts combined with optical image from Digitized Sky Survey allow us to conclude that RX J0046.4+4204is actually a distant galaxy cluster.In this paper,we assume the ΩM =0.3and ΩΛ=0.7cosmology with the Hubble constant of H 0=71km/s/Mpc.For the defined above cosmology and the measured redshift of z =0.290,the angular size of 1′corresponds the physical size of 257kpc.Statistical un-certainties are quoted at the 90%confidence level unless there is a statement saying otherwise.2.OBSERVATIONS AND DATA REDUCTIONIn the following analysis,we use the data from XMM-Newton observation of the XMM North 3Field of M31centered at RA =00h 46m 38s .00;Decl =+42◦16′20.0′′.Fig.1(Left )shows optical image of M31from Digitized Sky Survey with X MM-Newton FOV shown as a circle for M31North 3field.The XMM-Newton observation was performed on 2002June 29as a part of the Guar-anteed Time Program (PI:K.O.Mason).An analysis of the complete North 3field is presented in Trudolyubov2KOTOV,TRUDOLYUBOV,&VESTRAND42:35:59.959:59.941:23:59.947:59.940:11:59.9R.A. (J2000)D e c l . (J 2000)RXJ0046.4+4204XMM M31 North 347:45.616.80:46:48.019.250.445:21.625:11.920:59.916:47.942:12:35.908:23.904:11.9R.A. (J2000)D e c l . (J 2000)32541RXJ0046.4+4204Fig.1.—Left:Optical image of M31from Digitized Sky Survey with X MM-Newton FOV show as a circle for M31North 3field.Right:The combined MOS1-MOS2-PN vignetting-corrected image of the XMM North 3Field of M31in 0.8−2.5keV energy band,square root intensity scale.33.628.80:46:24.019.214.406:35.905:59.923.947.942:04:11.903:35.959.902:23.9R.A. (J2000)D e c l . (J 2000)Fig. 2.—The Palomar Digitized Sky Survey optical image with overlaid brightness contours made from 0.8-2.5keV band MOS2/PN image.The X-ray image was smoothed with a 4′′Gaus-sian kernel.The contour levels are based on the background noise going up in logarithmic steps.MOS1image was not used for con-struction of the contours because the center of the cluster falls at the edge of a CCD in MOS1camera.The angular size of the largest contour corresponds to ∼0.7Mpc at z =0.29.et al.(2005).In current analysis,we used the data from two EPIC-MOS detectors (Turner et al.2001)and the EPIC-PN detector (Str¨u der et al.2001).The EPIC data was re-duced using the standard XMM-Newton Science Analysis System (SAS v6.1.0)5.We used the calibration database with all updates available prior to January,2005.Only X-ray events corresponding to patterns 0-12for MOS de-tectors and patterns 0-4for PN detector were selected.All known bad pixels were excluded.5See http://xmm.vilspa.esa.es/external/xmm cal/sas.shtmlThe EPIC background is highly variable and only its quiescent component can be accurately modeled.To de-tect and exclude the periods of high flaring background,we produced the light curves for each EPIC detector showing count rate in the 2.0-15.0keV energy band from the whole field of view but with detected sources masked.The light curves were binned to 200s time resolution.We screened the EPIC data to recursively exclude time in-tervals with the deviation of the count rate exceeding a 2σthreshold from the average.Experiments with differ-ent choices of energy bands and flare detection thresh-olds have shown that our choice was close to optimal.The remaining good exposure time was ∼24ks for the EPIC-PN,∼41ks for the EPIC-MOS1,and ∼43ks for the EPIC-MOS2.To account for strong XMM mirror vignetting,we used an approach proposed by Arnaud et al.(2001).Each photon was assigned a weight proportional to inverse vi-gnetting and these weights were then used in computing images and spectra.This was done using the SAS tool evigweight .Background modeling in our analysis was implemented following the double-subtraction method of Arnaud et al.(2002).The first step of this method is to subtract the particle-induced background component.This compo-nent can be estimated from a set of XMM observations with the filter wheel closed (so called “closed data”).We compiled the closed dataset from public observations available in the XMM data archive;these data were re-duced following identical steps as the science observa-tions.The closed background was adjusted to the cluster observations using the observed flux in the 10–15keV band outside the field of view.The scaling factors are 1.04,1.05,and 1.01for MOS1,MOS2,and PN respec-tively.The second step is to determine the cosmic X-ray background (CXB)component.Its spatial distribution should be flat because vignetting correction is already ap-plied.Therefore,the CXB component can be measured in the source-free regions of the field of view at large radii from the cluster center (see §3.1and 3.2below).A GALAXY CLUSTER BEHIND M313TABLE1Results of Spatial binedEPIC-PN,MOS1and MOS2data,0.8−2.5keV energy range.Parameter errorsquoted are90%confidence limits.Parametersβfitα−βfitα···1.54±0.25β0.60±0.030.70±0.08r c(′′)20.2±2.856±16r c(kpc for z=0.290)87±12240±69χ2/dof156.7(119)139.4(118)Finally,we applied a correction for photons registered during the CCD readouts,so called out-of-time events, to the EPIC-PN data.3.RESULTSThe combined MOS1,MOS2,and PN image of the XMM North3Field of M31in the0.8−2.5keV en-ergy band,corrected for the effects of instrumental vi-gnetting,is shown in Figure1(Right).The raw image was convolved with a Gaussian function with spatial scale σ=4′′.We define the cluster center to be at the location of the X-ray surface brightness peak of the cluster emis-sion in the0.8−2.5keV energy band,α=00h46m24s.8δ=+42◦04′26′′(J2000),with an estimated uncertainty radius of6′′(90%CL),determined by the wavelet de-composition algorithm of Vikhlinin et al.(1998).The Palomar Digitized Sky Survey optical image shows no extended optical counterpart for RX J0046.4+4204(see Fig.2).During the following spectral and spatial analysis,we excluded all detectable point sources from the data.The sources were detected separately in the“optimal”0.3-3keV,“soft”0.3-0.8keV,and“hard”2.0-6.0keV energy bands using the task wvdecomp of the ZHTOOLS package 6.Detected point sources were masked with circles of 80%PSF power radii.3.1.Spatial AnalysisSpatial analysis of the cluster emission was performed in the0.8−2.5keV energy band using all detectors.Ex-periments with different energy bands showed that the signal to noise ratio was close to optimal for the chosen band.We used a pixel size of4′′in our spatial analysis. The image for each camera was corrected for vignetting. The PN image was corrected for out-of-time events.We subtracted the particle background component from the images as described in§2.We extracted the azimuthally averaged surface bright-ness profiles centered on the X-ray surface brightness peak,excluding the CCD gaps and circles around the point sources.The profiles were logarithmically binned with a step of∆r=0.1r.A logarithmic radial bin-ning approximately preserves the signal to noise ratio in annuli until the background becomes comparable to the signal.For the extracted profiles,the chosen step keeps the signal to noise ratio above3in annuli until∼5′.The obtained profiles were used to derive the parameters of 6See/∼alexey/zhtools/the spatial distribution of the ICM,clusterfluxes,and the level of the CXB component.The cluster surface brightness profiles are often mod-eled with the so calledβ-model,n2e∝(1+r2/r2c)−3βor S x∝(1+r2/r2c)−3β+0.5(Cavaliere&Fusco-Femiano 1976).However,this model poorly describes clusters with sharply peaked surface bigness profiles related to the radiative cooling of the ICM in the cluster centers. We used the simple modification of theβ-modeln2e∝(r/r c)−α4KOTOV,TRUDOLYUBOV,&VESTRAND1998).We estimated the count rate coming from the 70kpc central region using the bestfitα-βmodel.The total count rate was calculated integrating the observed surface brightness profile within1.4Mpc radius.We ob-tained f70kpc=18%.The combined MOS1-MOS2-PN surface brightness profile along with the bestfitα-βmodel are shown in Fig.3.From Fig.3one can see how the XMM PSF can flatten a peaked profile.For comparison,we alsofit the surface brightness profiles by the standardβ-model set-tingα=0.The obtained parameters are summarized in Table1.3.2.Global SpectrumFor our spectral analysis,we used the screened vignetting-corrected data in the0.5−10.0keV energy band from all cameras.The spectra of the cluster were extracted from a circular region with angular radius of 3.1′(0.8Mpc for z=0.290)for all EPIC data.All de-tected contaminating point-like sources were excluded from the source and background extraction regions.We subtracted the particle background component from the images as described in§2.To estimate the remaining CXB component,we extracted the spectra from a cir-cular region with angular radius of9.0′centered at the on-axis position,but a part of the region falling into the 9.65′(2.5Mpc for z=0.290)circle centered at the cluster center was excluded.The response matrices and effective areafiles were generated by the standard SAS tasks.Be-cause the data were previously vignetting corrected,the effective areafiles were created for the on-axis position using the routine arfgen.The response matrices were generated in the spectrum extraction region via rmfgen. The source spectra were binned to have at least30 counts in each spectral bin andfit in XSPEC11.3.0(Ar-naud1996)by the Mewe-Kaastra-Liedahl plasma emis-sion model(Mewe et al.1985).We used abundances from Anders&Grevesse(1989).Galactic photoelectric absorption was accounted for using the WABS model (Morrison&McCammon1983).The spectra from the EPIC-PN(3677counts),MOS1(1666counts)and MOS2 (2179counts)detectors werefitted both jointly and sep-arately.For the jointfits,only spectral model normaliza-tions were allowed to vary independently.The results of both joint and separate spectralfitting of the EPIC-PN, MOS1and MOS2data are summarized in Table2.We obtained a redshift value of0.290with2%accuracy. The redshift values estimated independently from EPIC-PN,MOS1and MOS2data are in good agreement within measurement errors.For the value of hydrogen column density we obtained N H=(2.2±0.20.2)×1021cm−2that is significantly above the Galactic hydrogen column in the direction of M31,∼7×1020cm−2(Dickey&Lockman 1990).We checked that the values of absorption obtained independently from EPIC-PN,MOS1and MOS2data are consistent within the measurement errors.The EPIC-PN,MOS1and MOS2spectra,along with the best-fit spectral models,are shown in Figure4.Note that separate spectralfitting of the EPIC-PN,MOS1and MOS2data gives consistent values of the model param-eters.3.3.Temperature profileTo construct temperature profile,we extracted indi-vidual spectra in5annuli:0−50kpc,50−100kpc, 100−200kpc,200−400kpc,and800−1600kpc,centered on the position of the X-ray surface brightness peak us-ing data from all cameras.After background subtraction, the number of counts(MOS1+MOS2+PN)in0.5-10kev energy band in1,2,3,4,and5annuli was632,1275, 2071,2069,1063,and459accordingly.The correction for the XMM PSF effect was done us-ing an approach of Pointecouteau et al.(2004).Using the best-fitα-βmodels of the cluster brightness and the XMM PSF calibration,we calculated the redistribution matrix,R ij,of each temperature to each annulus which represents relative contribution of emission from annulus i to the observedflux in annulus j.The model spectrum, S j,is then given byS j= R ij S(T i),(2) where T i is the temperature in annulus i and S(T i)is the mekal spectrum for this temperature.Fitting this model to the observed spectra in all annuli simultane-ously and treating all T i as free parameters gives the de-convolved temperature profile.Unfortunately,the statis-tic was poor to measure metallicity profile.So for all annuli wefixed the metallicity values at the best-fit val-ues obtained from the global spectrum.We checked that allowing the absorption and the metallicity to be freely fitted does not significantly change the result.The val-ues of redshift and absorption values were alsofixed at the best-fit values.Wefitted the observed temperature profiles by a3-D temperature model:T(r)=T0/(1.+(x/0.6)2)γ,(3) where x=r/r0.A similar model describes the tem-perature profiles for low redshift clusters(Vikhlinin et al.2005).For local clusters,r0scales with the clus-ter temperature as r0⋍0.50T1/2Mpc where T is in keV(see Fig.16in Vikhlinin et al.(2005)).For the best-fit temperature of T=5.5keV derived from the global spectrum,r0=1.17keV.Wefixed r0at the value suggested by the low redshift clusters only with an additional redshift scaling r0∝1/E(z),where E(z)=A GALAXY CLUSTER BEHIND M315Fig.3.—Left:Upper panel:The combined MOS1-MOS2-PN surface brightness profile of RX J0046.4+4204in the 0.8−2.5keV energy band.Black solid line shows α-βmodel best fit convolved with the XMM PSF.Black dashed line shows α-βmodel best fit.Black dotted line shows the XMM PSF.Black dotted-dashed line shows the level of the cosmic X-ray background component.Lower panel:The residual between the data and the best-fit model in terms of sigmas.Right:The same with the standard β-model bestfit.Fig. 4.—Left:Count spectra of RX J0046.4+4204taken with the XMM-Newton /EPIC-PN (black circles ),MOS1(blue triangles )and MOS2detectors (magenta squares ).The corresponding best-fit spectral models (absorbed red-shifted Mekal model)convolved with instrumental responses are shown as black ,red and blue solid lines.Right:Expanded view of the 4.0-7.0keV energy band.Upper,Middle and Lower panels show PN,MOS1and MOS2data.A red-shifted iron emission line feature is clearly evident.We applied Gaussian scatter to the observed tempera-ture profile within its uncertainties and fitted simulated profiles.The best-fit model uncertainties estimated as rms scatters in narrow radial bins from 1000simulations are shown in Fig.5..3.4.Mass and Luminosity measurementsAssuming hydrostatic equilibrium for the ICM,we can use the best fit temperature and density profiles to derive the total cluster masses:M (r )=−rT (r )d log r+d log T (r )6KOTOV,TRUDOLYUBOV,&VESTRANDTABLE 2Results of Spectral Analysis.EPIC-PN,MOS1and MOS2data,0.5−10.0keV energy range.Spectral extraction radius is 3.1′.Parameter errors quoted are 90%confidence limits .Parameters MOS1MOS2PNCombinedN H (1022cm −2)0.21±0.040.030.26±0.030.030.21±0.020.020.22±0.020.02kT (keV )6.4±1.41.15.3±0.80.75.1±0.70.55.5±0.50.5Z ⊙0.44±0.190.270.72±0.200.190.45±0.210.100.57±0.150.13z0.296±0.0110.0230.292±0.0090.0070.287±0.0080.0080.290±0.0050.005Fig.5.—Upper panel:Same as Fig.3(Left upper panel).Lowerpanel:The temperature profile of the cluster as a function of an-gular radius obtained from EPIC-PN/MOS1/MOS2data.Solid black circles show the deconvolved projected temperature profile.For comparison,open circles shows the raw measurement from the X-ray fit in the same annuli.The error bars correspond to 68%(1-σ)confidence limits.Solid line shows the best-fit projected tempera-ture profile and dashed lines correspond to its 68%CL uncertain-ties.1014M ⊙and the radius of r 500=1.09±0.08Mpc corre-sponding to the mean overdensity ∆=500relative to the critical density at the cluster redshift.The uncer-tainties on the total mass due to the temperature profile were calculated analogically to the uncertainties on the best-fit temperature profile model.We calculated the total mass for each simulated profile.Then the uncer-tainties were calculated as the boundaries of the region containing 90%of all realizations.The total mass un-certainties due to the error on the density gradient were calculated following Pratt &Arnaud (2002).The value of d log ρ(r )/d log r at r 500was considered as an indepen-dent parameter of the α-βmodel instead of β.We refit the surface brightness profile with the new parameter set and measured the uncertainties on d log ρ(r )/d log r at r 500.The final total mass uncertainties were calcu-lated by adding quadratically the total mass error due to the temperature profile and the density profile.The uncertainties on R 500are related to the total mass un-certainties as σM 500/M 500=3σr 500/r 500.We calculated the emission measure-weighted temper-atures (volume-averaged with weight w =ρ2g )of T emw =6.0±0.7within 70kpc <r <r 500.It is not a surprisethat obtained T emw is higher than the best-fit spectro-scopic temperature because we excluded the innermost bin from the temperature profile fit.The uncertainties for T emw were calculated from Monte-Carlo simulations in the similar way as for the temperature profile best-fit.We can compare the obtained M 500with predicted value from M −T relations.We will use as a low-redshift reference the M −T relation from Vikhlinin et al.(2005),which is similar to the M −T relation measured by Arnaud et al.(2005).The M −T relation derived by Vikhlinin et al.(2005)predicts M 500=6.1×1014M ⊙for a 6keV cluster.The self-similar theory predicts that for the same temperature the mass evolves as M ∆∝E (z )−1with z ,where E (z )=H (z )/H 0=(0.3(1+z )3+0.7)1/2(e.g.Bryan &Norman (1998)).Dividing the predicted mass by E (z =0.29)to place it at z =0.29,we obtain M 500=5.3×1014M ⊙.This value is in agreement with the derived M 500=(5.1±1.0)×1014M ⊙.We derived the unabsorbed bolometric luminosity ofL x =(8.4±0.5)×1044h −271erg s−1using the Mewe-Kaastra-Liedahl plasma emission model.We used T emw as the temperature parameter for the model.The model was normalized by the following count rate:we sub-tracted from the observed 0.8−2.5keV count rate cal-culated within 0<r <1.4Mpc the count rate calculated from the best-fit α-βmodel within r <70kpc,and mul-tiplied the result by 1.06(Markevitch 1998).The fact that our analysis is similar to the one done by Markevitch (1998)for low redshift clusters allows us to compare the obtained luminosity with the prediction from their L −T relation.The L −T relation derived by Markevitch (1998)predicts the luminosity of 6.2×1044h −271erg s−1for a 6keV cluster.It is well below the derived luminosity.However,correcting the predicted luminosity for the evolution in L −T relation,L z =(1+z )1.5L 0,reported by Vikhlinin et al.(2002),we obtain9.2×1044h −271erg s −1.This value is close to the observed one.4.DISCUSSION AND CONCLUSIONSOur deep XMM-Newton observations of M31have shown that RX J0046.4+4204is not located in that galaxy,but rather is actually a distant cluster of galax-ies.We found RX J0046.4+4204has spatially extended X-ray emission and that the spectrum clearly shows a red-shifted iron emission line.Straightforward fitting of the iron line yields a cluster redshift of Z=0.290with 2%accuracy and that the redshift values estimated in-dependently from EPIC-PN and MOS data are in good agreement within measurement errors.The large scale spatial distribution of RXA GALAXY CLUSTER BEHIND M317J0046.4+4204is well fit by the α-βmodel with β=0.70±0.8,a core radius r c =(56±16)′′or r c =(240±69)kpc for z =0.290,and α=1.54±0.25.The obtained values of βand r c are consistent with the parameters of typical clusters (Vikhlinin et al.1999).The derived αagrees with values measured for cooling flow clusters (Vikhlinin et al.2005).The spatially integrated X-ray continuum is well fit by red-shifted (z=0.290)Mewe-Kaastra-Liedahl plasma emission model with low energy photo-electric absorp-tion.The best fitting global model for the joint PN,MOS1,and MOS2measurements yields the parame-ters kT (keV )=5.5±0.5,fractional solar abundanceZ ⊙=0.57±0.150.13,,a redshift z =0.290±0.005,and a column depth n H =2.2(±0.20.2)×1021cm−2.This derived column depth is significantly larger than the Galactic hy-drogen column in the direction of M31,∼7×1020cm −2(Dickey &Lockman 1990).It is interesting to compare the value of absorption obtained for the cluster with absorption for the nearby X-ray sources.Fig 1(Right )shows five bright X-ray sources lying in the direct vicinity of RXJ0046.4+4204for which Trudolyubov et al.(2005)were able to measure column depth based on their X-ray spectra and to iden-tify some of them.XMMU J004540.5+420806(source #5in Fig.1)was identified as a foreground star with the column depth of 0.2×1021cm −2(two sigma upper limit).XMMU J004648.0+420851(source #3)was identified as a background radio source withthe column depth of (4.0±1.05.0)×1021cm−2.XMMU J004627.0+420151(source #1)was identified as a glob-ular cluster source in M31.The derived column depth for this source was (1.3±0.1)×1021cm −2.The nature of two last sources,XMMU J004611.5+420826(source #4)and XMMU J004703.6+420449(source #2),was unclear.Trudolyubov et al.(2005)proposed that these sources could be two AGN located in the back-ground of M31.The derived column depths of XMMU J004611.5+420826and XMMU J004703.6+420449were (2.5±0.3)×1021cm −2and (2.3±0.6)×1021cm −2,re-spectively.The absorption value of the background radio source is 2times higher than the cluster absorption and most likely to be intrinsic.The column depth of the glob-ular cluster candidate is smaller than that of the clus-ter.To explain this fact,it might be proposed that the globular cluster candidate is located in front of the disk of M31,while the cluster is obscured by the disk.On the other hand,XMMU J004611.5+420826and XMMU J004703.6+420449have column depths consistent with that of the cluster,suggesting that they could be also located in the background of M31,although it is unclear what fraction of the column depths is intrinsic.This in-terpretation is in general agreement with results of spec-tral fitting of a large sample of M31globular cluster X-ray sources (Trudolyubov &Priedhorsky 2004).Tru-dolyubov &Priedhorsky (2004)found that globular clus-ter sources located in front of M31disk have typical val-ues of absorbing column in the range of (0.5−1.5)×1021,while the sources located behind the disk or embedded into it show higher absorbing columns of (2−4)×1021cm −2.The extracted temperature profile corrected for the XMM PSF shows the central temperature decline that confirms the indication of cooling flow presence from the spatial ing the spatially resolve tem-perature profile we derived R 500=1.09±0.07Mpc,M 500=(5.3±1.0)×1014M ⊙,and T emw =6.0±0.7within R 500.The values of M 500corrected for the evolution and T emw are in agreement with local M −T relations.The study we have presented here shows the utility of sensitive X-ray observations for identifying and studying clusters of galaxies in directions where foreground confu-sion or heavy optical extinction makes optical selection complicated.We thank A.Vikhlinin for extensive discussions and helpful comments.Also we’d like to thank the referee for providing useful suggestions.This paper is based in part on observations obtained with XMM-Newton ,an ESA science mission with instruments and contributions directly funded by ESA Member States and the USA (NASA).This work was supported in part by NASA grant NAG5-12390and by Internal Laboratory Directed Research and Development funding at Los Alamos Na-tional Laboratory.REFERENCESArnaud,K.,1996,in Astronomical Data Analysis Software and Systems V,ASP Conference Series 101,ed.G.Jacoby &J.Barnes (San Francisco:ASP)17Anders,E.,&Grevesse,N.1989,Geochim.Cosmochim.Acta,53,197Arnaud,M.,Neumann,D.M.,Aghanim,N.,et al.,2001,A&A,365,L80Arnaud,M.,Majerowicz,S.,Lumb,D.,et al.,2002,A&A,390,27AArnaud,M.,Pointecouteau, E.,&Pratt,G.W.2005,A&A,submitted (astro-ph/0502210)Bryan,G.L.,&Norman,M.L.1998,ApJ,495,80Cavaliere,A.,Fusco-Femiano,R.,1976,A&A,49,137Dickey,J.M.&Lockman,F.J.1990,ARA&A,28,215Ebeling,H.,Mullis,C.R.,&Tully,R.B.2002,ApJ,580,774Evrard,A.E.1997,MNRAS,292,289Kirsch,M.,2005,EPIC Status of Calibration and Data Analyis,http://xmm.vilspa.esa.es/docs/documents/CAL-TN-0018-2-4.pdfMarkevitch,M.,1998,ApJ,504,27Mazzotta,P.,Rasia, E.,Moscardini,L.,&Tormen,G.2004,MNRAS,354,10Mewe,R.,Gronenschild,E.H.B.M.,&van den Oord,G.H.J.1985,A&AS,62,197Morrison,R.,&McCammon,D.1983,ApJ,270,119Nevalainen,J.,Lumb,D.,dos Santos,S.,Siddiqui,H.,Stewart,G.,and Parmar,2001,A.N.,A&A,374,66-72Peres,C.B.,Fabian,A.C.,Edge,A.C.,Allen,S.W.,Johnstone,R.M.,&White,D.A.1998,MNRAS,298,416Pointecouteau,E.,Arnaud,M.,Kaastra,J.,&de Plaa,J.2004,A&A,423,33Pratt,G.W.,Arnaud,M.,2002,A&A,394,375Press,W.H.,&Schechter,P.1974,ApJ,187,425Rosati,P.,Borgani,S.,&Norman,C.2002,ARA&A,40,539Sarazin,C.L.1988,Cambridge Astrophysics Series,Cambridge:Cambridge University Press,1988,Supper,R.,Hasinger,G.,Lewin,W.H.G.,et al.,2001,A&A,373,63Str¨u der,L.,et al.2001,A&A,365,L1Trudolyubov,S.,&Priedhorsky,W.2004,ApJ,616,821Trudolyubov,S.,Kotov,O.,Priedhorsky,W.,Cordova, F.,&Mason,K.2005,ApJ,634,314Turner,M.J.L.,et al.2001,A&A,365,L27Vikhlinin,A.,McNamara,B.R.,Forman,W.,Jones,C.,Quintana,H.,&Hornstrup,A.1998,ApJ,502,558Vikhlinin,A.,Forman,W.,&Jones,C.1999,ApJ,525,47Vikhlinin,A.,VanSpeybroeck,L.,Markevich,M.et al.,2002,ApJ 578,L107。
X-ray 辐射X射线英文PPT
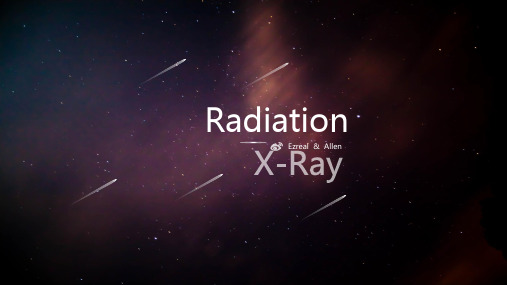
X - ray irradiation 射线的照射
Penetrating Action 穿透作用
Ionization Effect 电离作用
Biological response 生物反应
Application method Application examples 应 用 例
Measure 测量
Non-destructive Inspection 非破坏检查
・Concentration measurement 浓度计测 ・Density measurement 密度计测
・ X light perspective X光透视
・ CT detection CT检查
Diagnosis 诊断
・X - ray radiography X射线造影
Radiation
Ezreal & Allen
Vocabulary
X-ray:X射线 X-ray spectrum:射线谱 Coherent scattering:相干散射 Incoherent scattering:非相干散射 attenuation of X-ray: X射线的衰减 Absorb of X-ray: X射线的真吸收 Optic-electric effect:光电效应 fluorescent radiation:荧光辐射
h = Planck's constant = 1.38 10-16 erg sec
X-Ray Tubes
X-Ray Tubes
1-高压变压器;2-钨丝变压器; 3-X射线管;4-阳极; 5-阴极;6-电子;7-X射线
7 3
5
6
4 2
1
Application of X-ray
ASCA X-ray source catalogue in the Galactic Center region
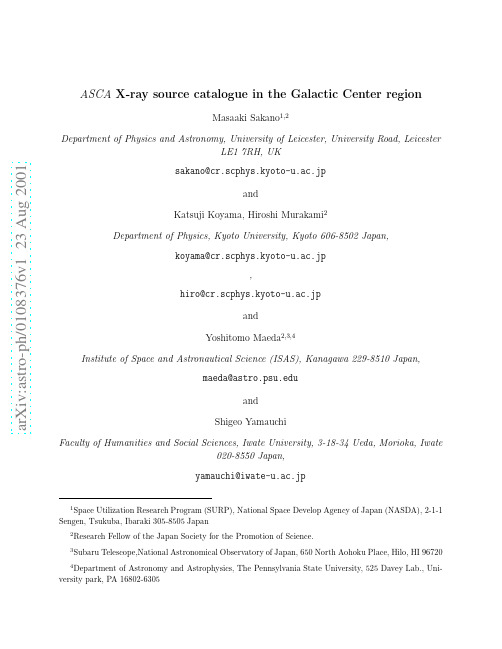
a rXiv:as tr o-ph/18376v123Aug21ASCA X-ray source catalogue in the Galactic Center region Masaaki Sakano 1,2Department of Physics and Astronomy,University of Leicester,University Road,Leicester LE17RH,UK sakano@cr.scphys.kyoto-u.ac.jp and Katsuji Koyama,Hiroshi Murakami 2Department of Physics,Kyoto University,Kyoto 606-8502Japan,koyama@cr.scphys.kyoto-u.ac.jp ,hiro@cr.scphys.kyoto-u.ac.jp and Yoshitomo Maeda 2,3,4Institute of Space and Astronautical Science (ISAS),Kanagawa 229-8510Japan,maeda@ and Shigeo YamauchiFaculty of Humanities and Social Sciences,Iwate University,3-18-34Ueda,Morioka,Iwate020-8550Japan,yamauchi@iwate-u.ac.jpABSTRACTThe ASCA satellite made107pointing observations on a5×5deg2region around the center of our Milky Way Galaxy(the Galactic Center)from1993to1999.In the X-ray images of the0.7–3keV or3–10keV bands,we found52point sources and a dozen diffuse sources.All the point sources are uni-formlyfitted with an absorbed power-law model.For selected bright sources,Sgr A∗,AX J1745.6−2901,A1742−294,SLX1744−300,GRO J1744−28,SLX1737−282,GRS1734−292,AX J1749.2−2725,KS1741−293,GRS1741.9−2853,and an unusualflare source XTE J1739−302,we present further detailed spec-tral and timing analyses,and discuss their nature.The dozen extended X-raysources comprise radio supernova remnants,giant molecular clouds,and somenew discoveries.Most show emission lines from either highly ionized atoms orlow-ionized irons.The X-ray spectra werefitted with either a thin thermal orpower-law model.This paper summarizes the results and provides the ASCAX-ray source catalogue in the Galactic Center region.Subject headings:catalogs—surveys—Galaxy:center—X-rays:general—X-rays:stars—stars:general1.INTRODUCTIONStars,hot gas,and cold gas are densely distributed near the center of galaxies,which may lead to various activities,e.g.,mass accretion on a massive black hole,starbursts,and supernova explosions.These phenomena are also suggested in the center of our Milky Way galaxy,the Galactic Center region.Its proximity to us makes the Galactic Center region an excellent laboratory for the most detailed study on such activity,hence it has been one of the major research objects(see reviews by Morris&Serabyn1996;Mezger,Duschl,&Zylka 1996).Hard X-rays are key wavelengths for the study of the Galactic Center activity because a putative massive black hole,starbursts,and their relics are all potential X-ray emitters,and those X-rays are almost free from interstellar absorption.Initially,hard X-ray studies have been made with non-imaging instruments(no mirror)(e.g.,Kellogg et al.1971;Proctor et al.1978;Skinner et al.1987;Kawai et al.1988;Yamauchi et al.1990;Sunyaev et al.1991b; Sunyaev,Markevitch,&Pavlinsky1993;Pavlinsky,Grebenev,&Sunyaev1994;Churazov et al.1994).The most distinct components in the Galactic Center region were variable point sources (e.g.,Pavlinsky et al.1994),which are binaries with black holes or neutron stars,and the Galactic nucleus.Other than the point sources,supernova remnants(SNRs)and largely extended high-temperature plasma were also found(e.g.,Koyama et al.1989).Most of the previous instruments,however,suffered from severe source-confusion problem in the crowded Galactic Center region.ASCA is thefirst satellite equipped with X-ray mirrors and detectors that have a high sensitivity and reasonable spectral and spatial resolutions in the wide energy band up to 10keV,hence has been used for more detailed imaging spectroscopy in the Galactic Center region(e.g.,Koyama et al.1996;Maeda&Koyama1996;Maeda1998;Tanaka et al.2000; Sakano2000).The next hard X-ray mirror satellite,Beppo-SAX,also revealed new high-energy aspects near the Galactic Center region(Sidoli et al.1999).These works,however,have been limited to individual X-ray sources.In order to obtain comprehensive and unbiased knowledge on the central5×5degree2region,we have per-formed a complete survey since1998with ASCA.This paper summarizes the results with the following order.Details for the observations and the data screening are given in§2. In§3,X-ray images of the Galactic Center region in the hard and soft energy bands are constructed.Section4provides the detected sources,their spectral parameters,and iden-tifications.Further details for selected bright sources are presented in§5.Throughout this paper,we assume the distance to the Galactic Center to be8.5kpc.2.OBSERVATIONSASCA has four X-ray telescopes(XRT)with focal plane detectors of two Solid State Imaging Spectrometers(SIS0and1)and two Gas Imaging Spectrometers(GIS2and3).In-strument details are found in Serlemitsos et al.(1995),Burke et al.(1991,1994),Yamashita et al.(1997),Ohashi et al.(1996),Makishima et al.(1996),while a general description of ASCA can be found in Tanaka,Inoue,&Holt(1994).Since thefield of view of SIS is smaller than that of GIS,and the performance of the SISs has been significantly degraded since1995due to the radiation of charged particles (Dotani et al.1997),this paper refers to the GIS data unless otherwise stated.The GISs were generally operated in the PH mode with the standard bit-assignment(10-8-8-5-0-0;see Ohashi et al.1996),unless otherwise noted(Table1).The GISfields at selected targets including4TOO(Target Of Opportunity)transient sources were observed with the pointing mode.In order tofill blankfields,we performedfollow-up survey observations from1998to1999.In total,the Galactic Center5◦×5◦region was observed107times from1993to1999.Table1and Figure1summarize the observation log with the sequence numbers(Obs-ID,here and after).The total exposure time in the Galactic Center5◦×5◦area is about1600ksec,however,the exposure for each GIS field scatters largely from5to200ksec,depending on the observation modes or objectives (targets).For the follow-up surveyfields,shorter exposures(e.g.,10ksec)were generally allocated,while longer exposures(e.g.,200ksec)were achieved for multiple-pointings on selected targetfields.Data screening was performed,using the standard method described in Day et al. (1995).The data with telemetry saturation of larger than2σlevel are discarded,except for Obs-ID27field(see Table1),where the telemetry saturation were corrected according to Nishiuchi et al.(1999).The rise-time discrimination was applied to reject non-X-ray background(NXB),except for Obs-ID=27(see Table1).For timing analyses,barycentric arrival time correction was made for all the X-ray photons.3.X-RAY IMAGESFigures2a and2b show the X-ray images in the Galactic Center5◦×5◦region with the energy bands of3.0–10.0and0.7–3.0keV,respectively.A blank region around(l II,b II)= (−1◦.5,−1◦.5)is due to unexpected accidents in the ASCA operation.A region around the brightest source GX3+1is heavily contaminated by the stray light(see next paragraph), hence is deleted as is seen by a blank semi-circle around(l II,b II)=(2◦.3,0◦.8).For the same reason,some pointing data for extremely bright transient sources,such as theflare data of GRO J1744−28,are discarded.In the hard energy band of3–10keV(Fig.2a),the brightest are X-ray point sources. Radial structures seen around(l II,b II)=(−0◦.2,−0◦.8)and(0◦.6,1◦.0)are artifacts made by the stray lights from the bright sources.Since the stray lights are time variable,some artificial discontinuity cannot be removed in the re-constructed image,or mosaic-image(e.g., Serlemitsos et al.1995).Ignoring the artificial structures around bright point sources,we still see further diffuse structures,in particular near the Galactic plane.These are local enhancements of the hot plasma and X-ray reflection nebulae(Koyama et al.1996;Maeda1998;Maeda et al.1999; Murakami et al.1999,2000a,2000b,2001;Sakano et al.1999c,2000a;Tanaka et al.2000).The X-ray image in the soft energy band(0.7–3keV;Fig.2b)is largely different from that of the hard energy band.The bright hard-band point sources,located near the Galacticplane,become fainter due to the heavy absorption(e.g.,Sakano et al.1999b;Sakano2000). Diffuse clumpy structures are clearer than that in the hard band.The stray light structures become dim,due simply to the reducedflux of the original point sources.4.X-RAY SOURCE LIST IN THE GALACTIC CENTER REGIONSince the diffuse background and stray light structures are complex,source detection cannot be a simple job.We therefore developed the source detection procedures following the method by Ueda et al.(1998,1999).Wefirst pick up source candidates from each pointing image.This procedure can resolve neighboring sources separated as small as1arcmin.We then determine the accurate source positions by a2-dimensional image-fitting of the raw GIS2+3image,using the relevant point spread function(PSF)and the background taken from the off-plane blank sky.The normalization of the background is allowed to be free.We apply thefitting to narrow regions with the size of10′×10′centered at the source to minimize the spatialfluctuation of the background in thefitting region.These sourcefinding procedures are applied separately to the soft-band(0.7–3.0keV)and the hard-band(3.0–10.0keV)images of each observation.Although the statistical errors of the positions are less than10′′,the systematic errors due to the backgroundfluctuation and the calibration uncertainty is far larger,particularly near the edge of the GISfield.Thus,the nominal positional error radius is50′′in90% confidence.For each of the candidate sources,we accumulate the spectrum from a circle with a radius of3′.The background spectrum is made from the annular region with radii of3′–5′centered at the source position.In these accumulations,we reject the pixels located within 2′from nearby source candidates or diffuse enhancements.We define the significance to be the ratio of the source counts to the1σfluctuation of the relevant background,and set the source detection criteria to be at the5σlevel.Above procedures occasionally picked up“false”sources from bright diffuse structures, either real diffuse or stray light,as candidates of a point source or superposition of point sources.We therefore inspected all the source images and removed the“false”candidates from the source catalogue.Each source spectrum wasfitted with an absorbed power-law function.When the spec-tral parameters were not constrained,we used the best-fit values obtained with the best-quality spectrum(for multiple pointing)orfixed them to the lower-limit or upper-limitvalues,and estimated the0.7–10keVflux.All the results are summarized in Table2.The source identification and the counterpart search were made,using the past references and the SIMBAD database.Thirty-one sources are found to have a counterpart.The results are listed in Table2.By the inspection of the X-ray image and discarding the stray light structures,we found a dozen diffuse X-ray sources.Most of them are X-rays from radio SNRs,but some are new discoveries.The spectra arefitted with an absorbed thin thermal plasma model or a power-law function(and emission lines).The best-fit parameters and other relevant information are summarized in Table3.5.NATURE OF SELECTED BRIGHT SOURCES5.1.Sgr A∗&AX J1745.6−2901ASCA has observed the Sgr A region four times(see Table2):twice in1993(Obs-ID=2,7),once in1994(Obs-ID=12)and in1997(Obs-ID=39).The observation span is thus3.5yrs,which is the longest monitoring of Sgr A ever achieved by hard X-ray imaging instruments.We thus study long-term behaviors of X-ray sources in the Sgr A region.Since Koyama et al.(1996)reported that the spatial structure near to Sgr A is highly complicated, we analyze the data taken with the SIS detector,of which the spatial resolution is better than that of GIS.The SIS data are available in three of the four observations(Obs-IDs of2, 12,and39).Since the detailed results about the1993and1994data were already reported by Maeda et al.(1996)and Koyama et al.(1996),we mainly concentrate on the1997data unless otherwise stated.Figure3shows an SIS image in1997for the3–10keV band.Two X-ray sources seen in the1993and1994data(Koyama et al.1996;Maeda et al.1996)also appeared in1997;the north-east source(here and after,the Sgr A diffuse)is slightly extended(∼a few arcmin),of which the spatial peak is consistent with Sgr A*,while the south-west source is a low mass X-ray binary AX J1745.6−2901,named by Kennea&Skinner(1996).The Sgr A diffuse showed noflux variation(see§5.1.2)while AX J1745.6−2901was variable;the persistent fluxes of AX J1745.6−2901in the2–10keV band were1.2,6.8,and1.5×10−11erg cm−2s−1 in1993,1994and1997,respectively.We thus found that AX J1745.6−2901was in the low-flux state in1993and1997,and in the high state in1994.Figure4summarizes the variabilities of both the sources.5.1.1.AX J1745.6−2901Maeda et al.(1996)discovered intensity dips with the interval of8.356±0.008hr due to the eclipse from this source in the1994data.We newly analyze the1997data.We extracted X-ray photons from a circular region with a radius of0′.8,and made a light curve,just the same as Maeda et al.(1996).Then,we folded the light curves with8.356hr,and found a possible dip also in1997(Fig.5).Note that non-detection of the dip in1993is probably due to its short exposure of17ksec,which is about one-quarter of that in1997(70ksec).To constrain the orbital period,we determined the center of the eclipsing phase to be MJD49610.2326(2)and MJD50523.275(1)in the1994and1997observations,respectively, by assuming a well-type light curve for the eclipse.The time interval between these two eclipse-centers is then913.043(1)days.When no change of the orbital period is assumed, this time interval should be P×N,where P is the orbital period and N is an integer.From the period of8.356±0.008hr constrained by Maeda et al.(1996),we derived more accurate orbital period to be either8.34782,8.35100,8.35419,8.35737,8.36056or8.36375hrs with each error of0.00001hr.5.1.2.The Sgr A DiffuseWe made the SIS spectra of the Sgr A diffuse for the three observations.All of the data in the1993observation(Obs-ID=2)were available,whereas those for the1994and 1997observations were not.For the1994observation,only the data during the eclipse phase of AX J1745.6−2901were used,because X-rays from AX J1745.6−2901in non-eclipse phase,of which theflux was an order of magnitude larger than that of the Sgr A diffuse, highly contaminated the Sgr A diffuse spectrum.For the1997observation,we used only the1-CCD-mode data,in which the energy resolution was much better than that in the 4-CCD-mode(Yamashita et al.1997).The effective exposure time is then reduced to be18, 2.5,37ksec for the1993,1994,and1997data,respectively.For all the three observations,the spectra of the Sgr A diffuse and the background were taken from the paired regions given in Figure3.By subtracting the background data, we removed spill-over X-rays from AX J1745.6−2901and another transient source GRO J1744−28only seen in1997.Figure6shows the background-subtracted spectrum in1993,which has by far the best quality in these observations.Wefitted the spectrum in1993with a model of bremsstrahlung and Gaussian lines.From the line energies,we can identify these lines as Kαtransitions of highly ionized ions of silicon,sulfur,argon,calcium,and iron atoms.We thenfittedthe spectrum again,fixing the center energies of each line to the theoretical values,and determined the linefluxes.The best-fit parameters are listed in Table4.Thus,we quantitatively confirm the existence of optically thin thermal plasma just surrounding Sgr A*,which was initially reported by Koyama et al.(1996).The best-fit electron temperature is as high as∼8keV,which is consistent with those reported by Koyama et al.(1996)and Sidoli&Mereghetti(1999).For the Galactic Center plasma extending over the1-degree square region,Koyama et al.(1996)reported the prominent6.4-and6.97-keV lines,which are Kαemission of cold and hydrogen-like iron,respectively.Therefore,we included these two lines in thefitting,but obtained no significant linefluxes(Table4).In order to studyflux variations of the Sgr A diffuse,wefitted the spectra taken in 1994and1997with the same model given in Table4,allowing only the global normalization factor to be adjusted.The model well reproduced the spectral shapes of the two spectra.The best-fitfluxes of the three observations were constant within the statistical error,∼2×10−11 erg s−1cm−2(2–10keV).Hence,theflux and the spectral shape of the Sgr A diffuse should be steady in the long time-span of the three observations(Fig.4).5.1.3.Sgr A*The Sgr A diffuse,peaked at Sgr A*,is dominated by diffuse emission.With the three observations,the Sgr A diffuse showed no time-variability,which suggests that Sgr A*emits no significant point-like X-ray;the conservative upper-limit of the X-rayflux of Sgr A*is F X,Sgr A∗<2×10−11erg cm−2s−1(2–10keV).The X-ray luminosity of Sgr A*corrected for the interstellar absorption of7×1022H cm−2is then calculated to be L X,Sgr A∗<3×1035 erg s−1(2–10keV).This upper limit is consistent with that measured with SAX(3×1035erg s−1;Sidoli&Mereghetti1999)and the positiveflux recently reported with Chandra(2×1033 erg s−1;Baganoffet al.2001).Many hard X-ray observations near to Sgr A*with the non-imaging instruments had been performed before ASCA observations(for a summary of these observations,see Maeda et al.1996).These observations suggested a long-term time-variability of Sgr A*with the 3–10keVflux ranging(1–16)×10−11erg s−1cm−2,which corresponds to the absorption-corrected luminosity of about(1–20)×1035erg s−1(Skinner et al.1987;Kawai et al.1988; Sunyaev et al.1991a).Our ASCA monitoring over four years found that the low mass X-ray binary AX J1745.6−2901is always present,which supports the argument by Maeda et al.(1996)that the X-rayfluxes previously reported with non-imaging instruments might have suffered from possible contamination of this source.If theflux-change were due toAX J1745.6−2901,the periodic eclipse should be detected when the Sgr A region is in high state.However,ART-P reported no eclipse in the high state(Sunyaev et al.1991a);and accordingly the variability would not be likely due to AX J1745.6−2901,but possibly due to Sgr A*(R.Sunyaev,private communication).In fact,thefirst Chandra observation reports a hint of variability for a smallflare from Sgr A*(Baganoffet al.2001)although theflux level is an order of1033erg s−1.Presence of the moderately large variability should be tested by future Chandra and XMM-Newton observations.5.2.A1742−294,SLX1744−300&GRO J1744−28We found11X-ray bursts from A1742−294.The X-ray features are characteristic to the type-I bursts,hence we confirmed the previous results(e.g.,Lewin et al.1976)We clearly resolved SLX1744−300from a nearby burster,SLX1744−299(Pavlinsky et al.1994)separated by3′.Although we found11bursts from SLX1744−300,no burst was detected from SLX1744−299.SLX1744−300is known to exhibit X-ray bursts with complicated profiles(Skinner et al.1990,Pavlinsky et al.1994).The detailed natures of the bursts,however,were not studied with ASCA due to the limited statistics.GRO J1744−28is a type-II burster and a0.47s pulsar with an orbital period of11.83 days(e.g.,Finger et al.1996;Kouveliotou et al.1996).Detailed natures with the ASCA observations on and before1997March are found in Nishiuchi et al.(1999).This source was observed again on1998September7(Obs-ID=55&56)with ASCA,but no significantflux was detected with the3σupper limit of2×10−12erg cm−2s−1.5.3.XTE J1739−302A transient source XTE J1739−302was discovered with XTE at(17h39m00s,−30◦16′.2 (J2000))on1997August12(Smith et al.1997,1998b).Although it was the brightest source in the Galactic Center region on August12(∼3.0×10−9erg cm−2s−1from2to25keV), it was below the detection limit on9days before and2days after theflare.The SAX/WFC observation made on1997September6(Smith et al.1998b)found noflux from this source.We detected this source again with ASCA on1999March11(Obs-ID=76).Figure7 shows the light curve in the2–10keV band.Twoflares,which started at around MJD 51248.319,were detected.The light curve was unusual;it showed noflux,then suddenlyflared up and reachedthe peak after200–250s,dropped to noflux level with the same time scale as theflare-rise (200–250s).The secondflare had the almost identical profile except the peakflux of about a half of that of thefirstflare.No significant difference in the light curves is found between the hard(4–10keV)and the soft(1–4keV)energy bands.We accumulated the spectrum only during theflare.The spectrum is wellfitted(χ2/dof =91.4/77)with an absorbed power-law function of a photon indexΓ=0.80+0.10and a−0.11×1022H cm−2.On the other hand,Smith et al. hydrogen column density N H=3.17+0.33−0.31(1998b)reported that the XTE spectrum on1997August12was well described with a thermal bremsstrahlung model of kT=12.4±0.3keV.Hence,the ASCA result of theflat spectrum is very different from the XTE result;we note that the power-law index obtained with ASCA corresponds to a temperature over100keV when a bremsstrahlung model is applied.The column density is also different from the XTE/PCA result of(5–6)×1022 H cm−2(Smith et al.1998b).Thus,the spectral shape may have largely changed between the XTE and the ASCA observations.We made the X-ray images in the pre-flare phase,and found no X-rayflux at the source position with the3σflux upper limit of9×10−13erg cm−2s−1in the2–10keV band, assuming the same spectral shape during theflare phase.Since the peakflux of thefirstflare is about2×10−9erg cm−2s−1,this source exhibited drasticflux-increase by more than three orders within a few hundred seconds.Theflat spectrum,highly variableflux,and absorption resemble those of transient pulsars.We accordingly searched for pulsation in time scales of 1–100s with FFT analysis,and epoch folding for time scales of100–1000s,but found no significant pulsation,which is consistent with the XTE result by Smith et al.(1998b).5.4.SLX1737−282The nature of SLX1737−282(Skinner et al.1987),whether or not this is a persistent source,has been unknown.We observed SLX1737−282(AX J1747.0−2818)five occasions from1996to1999,and always detected positiveflux.The position is determined to be(l II, b II)=(359◦.971,1◦.231)with an error radius of40′′,which is within the error radius of the Einstein measurement(Skinner et al.1987).Therefore,we identified AX J1747.0−2818as SLX1737−282.The2–10keVflux of SLX1737−282was measured to be(3.5–5.0)×10−11erg cm−2s−1 through thefive ASCA observations.Thus,SLX1737−282seems fairly stable in the long time span,but exhibited short time variability of103–104seconds with amplitude of100% in the ASCA observations.The spectral shape had been also stable through2.5-yr observations with a power-law function of a photon indexΓ=2.1–2.4and a hydrogen column density N H=(1.8–2.2)×1022 H cm−2.This column density implies that the distance of this source may be several kpc or farther.The absorption-corrected luminosity in the2–10keV band is(4–7)×1035erg s−1for the assumed distance of8.5kpc.The power-law index,moderate variability,and the luminosity suggest that this source is a neutron star binary,although the other possibilities,such as an AGN,are not totally excluded.5.5.GRS1734−292GRS1734−292was discovered with Granat/ART-P in1990by Sunyaev(1990b).Since then,it has been observed in the wide energy band,from the soft X-ray band below2keV (Barret&Grindlay1996)to the hard X-ray band(Pavlinsky et al.1994),even up to400keV (Churazov et al.1992).Mart´ıet al.(1998)found this source to be a radio jet-like source, NVSS J173728−290802,and further identified it to be a Seyfert1galaxy with z=0.0214, combining the radio,optical,infrared,and X-ray results.With ASCA,the X-ray emission from this source has been always clearly detected whenever we observed this source;accordingly,GRS1734−292is probably a persistent X-ray source.The X-ray spectrum was well represented with a power-law function of a photon index of1.3–1.7and a hydrogen column density N H=(1.3–2.0)×1022H cm−2.The power-law index of1.3–1.7is within the nominal range of that of Seyfert1s.The column density of(1.3–2.0)×1022H cm−2is significantly smaller than N H∼6×1022H cm−2 determined with Granat/ART-P(Pavlinsky et al.1994),but is consistent with the result of Mart´ıet al.(1998),who estimated N H=(1.0±0.2)×1022H cm−2based on the measurement of optical extinction and several absorption lines.Therefore,our results support the Seyfert1 identification by Mart´ıet al.(1998).5.6.AX J1749.2−2725This source was discovered with ASCA in1995.Torii et al.(1998)found coherent pulsations of P=220.38±0.20s and P=220.44±0.80s periods in the Obs-ID=19,and Obs-ID=23&24observations,respectively.The energy spectrum was described with aflat power-law function(photon index ofΓ=0.7–1.3)with heavy absorption(N H=(7–13)×1022 H cm−2)through the ASCA observations until1997.We analyzed the data of Obs-ID=46in1998March and confirmed the pulsations of P=219.9±1.0s period.Since the error of the pulse period was large,we found no significant˙P from the previous observations.The spectral parameters except theflux were also consistent in each observation.Theflux of1×10−11erg cm−2s−1was a few times smaller than that in1995March(Obs-ID=19)and September(Obs-ID=23&24),but a few times larger than that in1996September(Obs-ID=33&34)and1997September(Obs-ID=43).5.7.KS1741−293&GRS1741.9−2853KS1741−293and GRS1741.9−2853are X-ray bursters(in’t Zand et al.1991;Cocchi et al.1999a).ASCA found two sources at the position of(l II,b II)=(359◦.5600,−0◦.0825) and(359◦.9507,0◦.1126)with the error radii of40′′.KS1741−293and GRS1741.9−2853lie within these error circles(e.g.,in’t Zand et al.1991,1998;Mandrou1990;Sunyaev1990a; Pavlinsky et al.1994).Although these two are not persistent sources and although we found no burst from both the sources,the positional coincidence,the apparent brightness in their high states, and the spectral parameters strongly favor that AX J1744.8−2921and AX J1745.0−2855 (Table2)are identical with KS1741−293and GRS1741.9−2853,respectively.We found that both the sources have showed apparent variability by a factor of50or more.Thefluxes in the quiescent state were less than a few10−12erg cm−2s−1in the0.7–10keV band(see Table2).The authors express their thanks to the ASCA team,and particularly,the ASCA Galac-tic plane/center survey team.We are grateful to Dr.K.Torii and an anomymous referee for their valuable comments and suggestions.They appreciate the help of Dr.Y.Ueda,Dr. Y.Ishisaki,Mr.J.Yokogawa,and Dr.L.Angelini in the analysis.We acknowledge Mr.A.Hands for correcting English.M.S.thanks Prof.K.Nishikawa,Dr.H.Awaki,and Dr. T.Tsuru.M.S.,H.M.,and Y.M.arefinancially supported by the Japan Society for the Promotion of Science for Young Scientists.A part of this work was based on the data in the SIMBAD database.REFERENCESBaganoff,F.,et al.2001,ApJ,in press(astro-ph/0102151)Bamba,A.,Yokogawa,J.,Sakano,M.,&Koyama,K.2000,PASJ,52,259Barret,D.,&Grindlay,J.E.1996,A&A,311,239Bouchet,L.,et al.1991,ApJ,383,L45Burke,B.E.,Mountain,R.W.,Daniels,P.J.,&Dolat,V.S.1994,IEEE Trans.Nuc.Sci.,41,375 Burke,B.E.,Mountain,R.W.,Harrison,D.C.,Bautz,M.W.,Doty,J.P.,Ricker,G.R.,&Daniels,P.J.1991,IEEE Trans.,ED-38,1069Churazov,E.,Gilfanov,M.,Cordier,B.,&Schmitz-Fraysse,M.C.1992,IAU Circ.5623Churazov,E.,Gilfanov,M.,&Sunyaev,R.1996,ApJ,464,L71Churazov,E.,et al.1994,ApJS,92,381Churazov,E.,et al.1995,ApJ,443,341Cocchi,M.,Bazzano,A.,Natalucci,L.,Ubertini,P.,Heise,J.,Muller,J.M.,&in’t Zand,J.J.M.1999a, A&A,346,L45Cocchi,M.,in’t Zand,J.J.M.,Bazzano,A.,Heise,J.,Muller,J.M.,Natalucci,L.,&Ubertini,P.1999b, Nucl.Phys.B,69/1,232Cremonesi,D.I.,Mereghetti,S.,Sidoli,L.,&Israel,G.L.1999,A&A,345,826Cui,W.,Heindl,W.A.,Swank,J.H.,Smith,D.M.,Morgan,E.H.,Remillard,R.,&Marshall,F.E.1997, ApJ,487,L73David,P.,Goldwurm,A.,Murakami,T.,Paul,J.,Laurent,P.,&Goldoni,P.1997,A&A,322,229Day,C.,Arnaud,K.,Ebisawa,K.,Gotthelf,E.,Ingham,J.,Mukai,K.,&White,N.1995,the ABC guide to ASCA data reduction,NASA/GSFCDotani,T.,Fujimoto,R.,Nagase,F.,&Inoue,H.1995,IAU Circ.6241Dotani,T.,Yamashita,A.,Ezuka,H.,Takahashi,K.,Crew,G.,Mukai,K.,&the SIS Team1997,The ASCA news(Greenbelt:NASA GSFC),5,14Elvis,M.,Plummer,D.,Schachter,J.,&Fabbiano,G.1992,ApJS,80,257Falcke,H.,Cotera,A.,Duschl,W.J.,Melia,F.,&Rieke,M.J.1999,“The central parsecs of the Galaxy”, ASP Conf.Ser.186,Finger,M.H.,Koh,D.T.,Nelson,R.W.,Prince,T.A.,Vaughan,B.A.,&Wilson,R.B.1996,Nature, 381,291Ghez,A.M.,Klein,B.L.,Morris,M.,&Becklin,E.E.1998,ApJ,509,678Goldwurm,A.,et al.1994,Nature,371,589Gray,A.D.1994,MNRAS,270,835。
X-rayemissionfrommassivestarswithmagneticfields
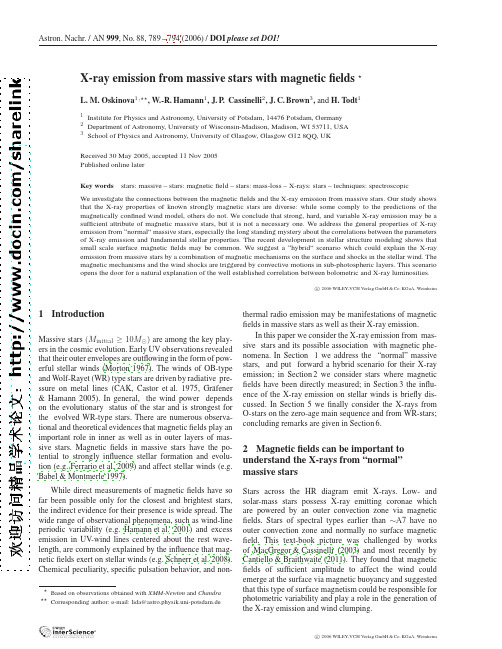
arX iv:111.5345v1[astro-ph.S R]24Oct211Astron.Nachr./AN 999,No.88,789–794(2006)/DOI please set DOI!⋆Based on observations obtained with XMM-Newton and Chandra⋆⋆Corresponding author:e-mail:*****************.uni-potsdam.de thermal radio emission may be manifestations of magnetic fields in massive stars as well as their X-ray emission.In this paper we consider the X-ray emission from mas-sive stars and its possible association with magnetic phe-nomena.In Section 1we address the “normal”massive stars,and put forward a hybrid scenario for their X-ray emission;in Section 2we consider stars where magnetic fields have been directly measured;in Section 3the influ-ence of the X-ray emission on stellar winds is briefly dis-cussed.In Section 5we finally consider the X-rays from O-stars on the zero-age main sequence and from WR-stars;concluding remarks are given in Section 6.2Magnetic fields can be important to understand the X-rays from “normal”massive stars Stars across the HR diagram emit X-rays.Low-and solar-mass stars possess X-ray emitting coronae which are powered by an outer convection zone via magnetic fields.Stars of spectral types earlier than ∼A7have no outer convection zone and normally no surface magnetic field.This text-book picture was challenged by works of MacGregor &Cassinelli (2003)and most recently by Cantiello &Braithwaite (2011).They found that magnetic fields of sufficient amplitude to affect the wind could emerge at the surface via magnetic buoyancy and suggested that this type of surface magnetism could be responsible for photometric variability and play a role in the generation ofthe X-ray emission and wind clumping.c 2006WILEY-VCH Verlag GmbH &Co.KGaA,Weinheim790L.M.Oskinova et al..:X-rays from massive stars with magneticfieldsM∗R−1∗,the velocity jump,i.e.the temperatures ofthe gas heated in the shock could be higher for more massivestars with higher T eff.This could explain the correlationsbetween T X and T effdiscovered by Walborn et al.(2009).3Magneticfields on massive stars are notnecessarily manifested via strong,hard andvariable X-raysFrom the small-scale magneticfields which we consideredin previous section,we now turn to the large-scale orga-nizedfields that may be fossil in origin.Babel&Montmerle(1997)studied the case of a star with a stellar wind and adipole magneticfield.They predicted that a collision be-tween the wind components from the two hemispheres inthe closed magnetosphere leads to a strong shock and char-acteristic X-ray emission.Based on this magnetically con-fined wind shock model(MCWS),the presence of a mag-neticfield on the O-type starθ1Ori C had been postulated.Direct confirmation of the magneticfield in this star byDonati et al.(2006a)proved that X-rays have large diagnos-tic potential in selecting massive stars with surface magneticfields.Using the parameters ofθ1Ori C,ud-Doula&Owocki(2002)and Gagn´e et al.(2005)performed MHD simula-c 2006WILEY-VCH Verlag GmbH&Co.KGaA,Weinheim Astron.Nachr./AN(2006)791792L.M.Oskinova et al..:X-rays from massive stars with magneticfieldsAstron.Nachr./AN(2006)793 Name Age Sp.Type log(L X/L bol)Presence of hard emission log(L X/L bol) Ref.Myr earliest O-type star earliest O-type star kT X>∼2keV ensemble of O stars(1)Hofner et al.(2002);(2)Rho et al.(2004);(3)Wolk et al.(2006);(4)Moffat et al.(2002);(5)L X is inferred using archival Chandra data;(6)Feigelson et al.(2002);(7)Cluster parameters from from Bonatto et al.(2006);other hand,some stars are weak X-ray sources,e.g.,Her36 (O7V)in the Hourglass and HD164492A(O7.5III)in the Trifid.Her36is of similar age and spectral type asθ1Ori C, yet the latter is significantly more X-ray luminous.The hardness of the X-ray spectrum is not an unambigu-ous indication of the presence of a magneticfield.X-ray temperatures of several keV are expected and have been ob-served in binary systems.The probability that a massive star in a young cluster is a binary is high,therefore it is not sur-prising that many stars listed in Table1display the presence of kT X>∼2keV plasma in their spectra.This is likely the case of the Kleimann star,a massive O4+O4binary ionizing the Omega Nebula cluster NGC6618.While its spectrum is rel-atively hard,the broad-band X-ray luminosity is consistent with the canonical L X≈10−7L bol relation which holds also for binaries(Oskinova2005).The X-ray observations of the Trifid Nebula(Rho et al. 2004)allowed to resolve the central ionizing source into discrete components and revealed that an O7.5III star has a soft(kT≈0.6keV)spectrum,while harder emission (kT≈6keV)is associated with a B-type star which is blen-ded with an unidentified source.Interestingly,X-rayflares were previously detected from B-type stars in two Orionis clusters:Trapezium andσOri(Sanz-Forcada et al.2004; Stelzer et al.2002).Often,young SFRs where massive stars are just forming are located close to more evolved massive star clusters,sug-gesting a possible causal connection between massive star feedback and star formation.An example of such SFR is ON2located close to the massive star cluster Berkeley87. Its X-ray properties were explored in Oskinova et al.(2010).The most evolved star in Berkeley87is a rare WO-type star,WR142.Stars of this spectral type are the ulti-mately latest evolutionary stage of a very massive stars(e.g. Sander et al.2011).There are no direct measurements of magneticfields on WR stars up to now(see Kholtygin et al., these proceed.).However,the X-ray properties of WR stars may provide indirect evidence that magnetism plays a role also in the latest stages of stellar evolution.Our XMM-Newton observation of the closest WO-type star WR142succeeded in detecting this object (Oskinova et al.2009).It was also detected by the Chandra observatory(Sokal et al.2010).Albeit the signal-to-noise of the observed spectrum was too poor for a detailed spec-tral analysis,from the hardness ratio Oskinova et al.(2009) concluded that the X-ray emission from this star is strongly absorbed in the wind,and is too hard to be explained by the wind-shock mechanism.We speculated that the hypo-thetical magnetic magneticfield can be responsible for this strong emission.Our analysis of the optical spectrum of this star yielded the fundamental stellar parameters:R∗=0.5R⊙,T eff= 160kK,M∗=5M⊙.Remarkedly,the optical emission line profiles in the spectrum of WR142have an unusual round shape.The formal rotational broadening models of these lines yield projected rotational velocity v sin i= 4000km s−1.Thus,the star may be rotating at break-up ve-locity.These stellar parameters compare well with those ex-pected for a SN orγ-ray burst progenitor(Paczy`n ski1998). 6Concluding remarksSurface magneticfields on massive stars are both predicted theoretically and confirmed observationally.All assive stars emit X-ray radiation,but the connection between X-rays and magnetism in massive stars is not yet fully understood. The MCWS model can explain the strong,hard,varable X-ray emission from some magnetic dipoles.However there is no quantitative explaination for the soft and the constant X-ray emission that is observed in the majority of magnetic massive stars.It was recently argued that all massive stars may have magneticfields on their surface(Cantiello&Braithwaite 2011).In this paper we propose a new scenario of X-ray emission from a massive star,where the plasma on the sur-face is heated by magnetic mechanisms while the plasma embedded in the stellar wind is heated by wind shocks.Both c 2006WILEY-VCH Verlag GmbH&Co.KGaA,Weinheim794L.M.Oskinova et al..:X-rays from massive stars with magneticfields。
纹理物体缺陷的视觉检测算法研究--优秀毕业论文
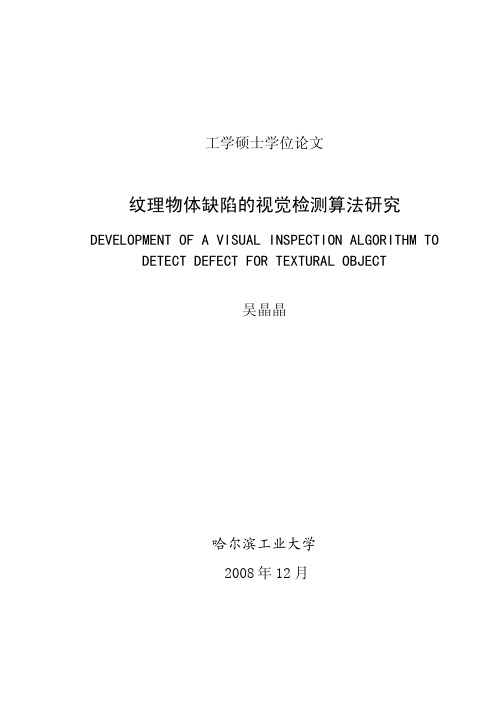
摘 要
在竞争激烈的工业自动化生产过程中,机器视觉对产品质量的把关起着举足 轻重的作用,机器视觉在缺陷检测技术方面的应用也逐渐普遍起来。与常规的检 测技术相比,自动化的视觉检测系统更加经济、快捷、高效与 安全。纹理物体在 工业生产中广泛存在,像用于半导体装配和封装底板和发光二极管,现代 化电子 系统中的印制电路板,以及纺织行业中的布匹和织物等都可认为是含有纹理特征 的物体。本论文主要致力于纹理物体的缺陷检测技术研究,为纹理物体的自动化 检测提供高效而可靠的检测算法。 纹理是描述图像内容的重要特征,纹理分析也已经被成功的应用与纹理分割 和纹理分类当中。本研究提出了一种基于纹理分析技术和参考比较方式的缺陷检 测算法。这种算法能容忍物体变形引起的图像配准误差,对纹理的影响也具有鲁 棒性。本算法旨在为检测出的缺陷区域提供丰富而重要的物理意义,如缺陷区域 的大小、形状、亮度对比度及空间分布等。同时,在参考图像可行的情况下,本 算法可用于同质纹理物体和非同质纹理物体的检测,对非纹理物体 的检测也可取 得不错的效果。 在整个检测过程中,我们采用了可调控金字塔的纹理分析和重构技术。与传 统的小波纹理分析技术不同,我们在小波域中加入处理物体变形和纹理影响的容 忍度控制算法,来实现容忍物体变形和对纹理影响鲁棒的目的。最后可调控金字 塔的重构保证了缺陷区域物理意义恢复的准确性。实验阶段,我们检测了一系列 具有实际应用价值的图像。实验结果表明 本文提出的纹理物体缺陷检测算法具有 高效性和易于实现性。 关键字: 缺陷检测;纹理;物体变形;可调控金字塔;重构
Keywords: defect detection, texture, object distortion, steerable pyramid, reconstruction
II
X-ray Emission from Elliptical Galaxies
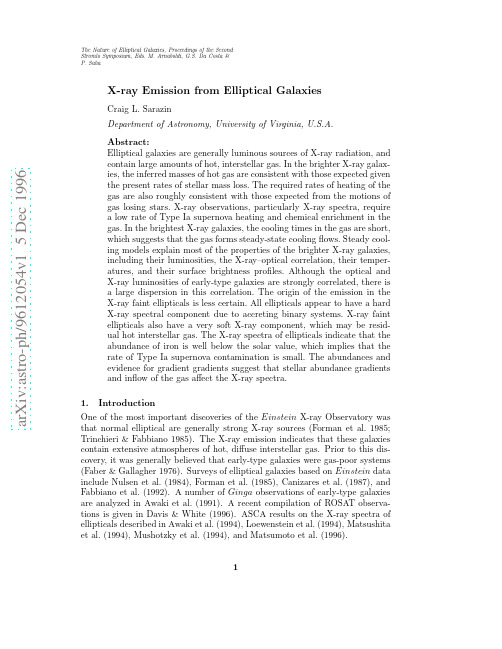
a r X i v :a s t r o -p h /9612054v 1 5 D e c 1996The Nature of Elliptical Galaxies,Proceedings of the SecondStromlo Symposium,Eds.M.Arnaboldi,G.S.Da Costa &P.SahaX-ray Emission from Elliptical GalaxiesCraig L.SarazinDepartment of Astronomy,University of Virginia,U.S.A.Abstract:Elliptical galaxies are generally luminous sources of X-ray radiation,and contain large amounts of hot,interstellar gas.In the brighter X-ray galax-ies,the inferred masses of hot gas are consistent with those expected giventhe present rates of stellar mass loss.The required rates of heating of the gas are also roughly consistent with those expected from the motions of gas losing stars.X-ray observations,particularly X-ray spectra,require a low rate of Type Ia supernova heating and chemical enrichment in the gas.In the brightest X-ray galaxies,the cooling times in the gas are short,which suggests that the gas forms steady-state cooling flows.Steady cool-ing models explain most of the properties of the brighter X-ray galaxies,including their luminosities,the X-ray–optical correlation,their temper-atures,and their surface brightness profiles.Although the optical and X-ray luminosities of early-type galaxies are strongly correlated,there is a large dispersion in this correlation.The origin of the emission in the X-ray faint ellipticals is less certain.All ellipticals appear to have a hard X-ray spectral component due to accreting binary systems.X-ray faint ellipticals also have a very soft X-ray component,which may be resid-ual hot interstellar gas.The X-ray spectra of ellipticals indicate that the abundance of iron is well below the solar value,which implies that the rate of Type Ia supernova contamination is small.The abundances and evidence for gradient gradients suggest that stellar abundance gradientsand inflow of the gas affect the X-ray spectra.1.IntroductionOne of the most important discoveries of the Einstein X-ray Observatory wasthat normal elliptical are generally strong X-ray sources (Forman et al.1985;Trinchieri &Fabbiano 1985).The X-ray emission indicates that these galaxies contain extensive atmospheres of hot,diffuse interstellar gas.Prior to this dis-covery,it was generally believed that early-type galaxies were gas-poor systems (Faber &Gallagher 1976).Surveys of elliptical galaxies based on Einstein data include Nulsen et al.(1984),Forman et al.(1985),Canizares et al.(1987),and Fabbiano et al.(1992).A number of Ginga observations of early-type galaxies are analyzed in Awaki et al.(1991).A recent compilation of ROSAT observa-tions is given in Davis &White (1996).ASCA results on the X-ray spectra of ellipticals described in Awaki et al.(1994),Loewenstein et al.(1994),Matsushita et al.(1994),Mushotzky et al.(1994),and Matsumoto et al.(1996).12Sarazin 2.X-ray PropertiesElliptical galaxies have X-ray luminosities which range over L X ≈1039−1042erg s −1.(All comparisons to observations in this paper assume a Hubble constant H o =50km/s/Mpc,and a distance to the center of the Virgo cluster of 25Mpc.)There appears to be a strong correlation between the X-ray and blue optical luminosities of early-type galaxies,with L X ∝L 1.6−2.3B .Figure 1shows the X-ray and optical luminosities of the early-type galaxies in the survey of Canizares et al.(1987).The filled circles are detections,while the inverted triangles are upper limits on L X .Obviously,there is considerable scatter about this relation.The X-ray emission from ellipticals is extended,with typical overall sizes of R X ∼50kpc.For example,Figure 2shows the ROSAT X-ray image of NGC 4472from Irwin &Sarazin (1996).On large scales,the azimuthally-averaged X-ray surface brightnesses I X (r )of ellipticals typically decline with radius r roughly as I X ∝r −2(Forman et al.1985).Very crudely,the X-ray and optical surface brightnesses of ellipticals decline in proportion to one another I X (r )∝I B (r )(Trinchieri et al.1986).This relationship holds only very approximately;the X-ray surface brightness profiles of ellipticals often have structure which is not apparent in the optical profiles.Also,this proportionality holds only within a given elliptical.The constant of proportionality varies from galaxy to galaxy,as required by the steeper than linear correlation between X-ray and opticalluminosities.91011123839404142Figure 1.The correlation of X-ray and optical luminosities of early-type galaxies from Canizares et al.(1987).The filled circles are detections,and the inverted triangles are upper limits.The hatched area gives the range of estimated stellar X-ray luminosities from Forman et al.(1985),Canizares et al.(1987),and Fabbiano et al.(1989).The curve gives the predicted relation for the steady cooling models of Sarazin &Ashe (1989).X-ray Emission3 At least for the more luminous X-ray ellipticals,spectral observations indi-cate that the X-rays are produced by thermal emission from diffuse gas.The spectra imply that the temperature of the gas is typically T≈107K(kT≈1 keV)(Forman et al.1985;Trinchieri et al.1986).The X-ray surface brightnesses profiles of ellipticals then indicate that the hot gas density declines with radius at large radii roughly asρgas∝r−3/2.The total gas masses required by the X-ray observations are rather uncertain,because this density distribution doesn’t converge and the X-ray images of ellipticals fade into the background at large radii.However,the gas masses are often in the range M gas≈109−1011M⊙.It is useful to compare the gas masses to the optical luminosities of the galaxies. For the brighter ellipticals,onefinds(M gas/M⊙)≈0.2(L B/L⊙).If a stellar mass to light ratio of≈10M⊙/L⊙is assumed,then is implies that the gas mass is about2%of the stellar mass.The hot gas is generally the dominant form of interstellar matter in elliptical galaxies(by mass).The interstellar gas masses in brighter ellipticals are large,but represent a much smaller fraction of the stellar mass than in late-type spiral galaxies.Figure2.The ROSAT X-ray image of the Virgo elliptical NGC4472(Irwin&Sarazin1996). Contours of the X-ray emission are superposed on a greyscale representation of the optical image.3.Physical State of the Gas3.1Origin of the GasThe amount of hot gas seen in the brighter X-ray ellipticals is consistent with the amount expected for the present rates of stellar mass loss acting over a significant fraction of the Hubble time.The present rate of stellar mass loss in early-type galaxies is fairly well-determined by stellar evolution theory to be4Sarazin(˙M∗/L B)≈1.5×10−11M⊙yr−1L−1⊙(Renzini&Buzzoni1986).If all of the stellar mass loss is converted into hot gas and the same rates have applied for a time t,the ratio of the mass of hot gas to the optical luminosity would now be(M gas/M⊙)≈0.16(L B/L⊙)(t/1010yr),which is quite close to the typical observed value for bright ellipticals.There are several caveats that need to be given concerning this agreement of the observed and predicted gas masses.First,models for the stellar evolution of elliptical galaxies predict that the stellar mass loss rate was much higher in the past.This and the fact that the gas in clusters of galaxies contains a very large mass of heavy elements suggest that elliptical galaxies have lost substantial amounts of gas in the past.Second,the agreement of the present stellar mass lost rate and the amount of hot gas really only applies to the brightest X-ray galaxies.For the fainter X-ray galaxies in Figure1,the masses of hot gas are apparently much smaller than from the present rates of stellar mass loss.Third, it is not obvious that all the gas resulting from stellar mass loss will be heated to X-ray emitting temperatures.Finally,elliptical galaxies are seldom very isolated, and large ellipticals near the centers of groups or poor clusters may be accreting ambient gas(Thomas1986).3.2Heating of the GasWhy is the interstellar gas in ellipticals hot?The primary reason is that the stars in elliptical galaxies move at large velocities relative to nearby stars.Thus, gas which is ejected from one star(for example,in a red giant wind or plane-tary nebula)will encounter gas ejected from other stars at a very high velocity, determined by the stellar velocity dispersion of the galaxy.Collisions between the ejected gas and either gas ejected by neighboring stars or ambient hot gas may thermalize the kinetic energy of motion of the gas.Because this gives an amount of heat which is proportional to the amount of gas injected,it is useful to define the energy per unit injected gas mass as3kT inj/2µm p.The resulting heating due to stellar mass loss is thenT inj=µm pσ2∗300km s−1 2,(1)whereσ∗is the one-dimensional stellar velocity dispersion,andµis the mean mass per particle in the gas in units of the proton mass m p.This gives a temperature which is comparable to those observed in ellipticals.If the gas in ellipticals forms a coolingflow,there will be additional heating due to infall in the galactic potential and adiabatic compression.Since the stellar velocity dispersion measures the depth of the potential well in an elliptical galaxy,this source of heating is also proportional toσ2∗,and can be viewed as a proportionate increase in equation1.In addition to the relatively quiescent stellar mass loss which dominates the injection of mass into the interstellar gas,a small fraction of the gas may be ejected with large kinetic energy by Type Ia supernovae.The heating rate perX-ray Emission5 unit injected gas mass due to supernovae isT inj=3.6×107K r SN1051ergs ˙M∗/L B21Λ(θ),(3)whereθ≡kT/(µm p).In general,the cooling time increases with radius in an elliptical galaxy.However,for the brighter X-ray ellipticals,the cooling time is shorter than1010yr over essentially all of the galaxy(see Table1in Sarazin [1990]).The major sources of heating of the gas are associated with its injection (equations1and2above),and cannot balance continuous cooling.Thus,one expects the gas to cool,and approach steady-state cooling over most of the observed regions of the brighter galaxies.6Sarazin4.Evolution of the Gas in EllipticalsThere are several reasons why the interstellar gas in ellipticals should evolve over time.First,the time scales for cooling and inflow become longer than the age at large radii,and the gasflows there are presumably time dependent.Second, the rates of stellar mass loss and supernova heating vary with time.Spherically symmetric,time-dependent hydrodynamical simulations of el-liptical galaxies have been made by a number of authors,including Loewenstein &Mathews(1987),D’Ercole et al.(1989),and David et al.(1990).Most of these models assume that elliptical galaxies form in a single short burst of star formation.Many of the models follow the evolution only after this burst.The most important characteristic of these models tends to be the rate of variation of the supernova heating rate compared to the total rate of stellar mass loss.In general,most models have a supernova rate which was higher in the past;this is particularly true if one includes the initial burst of star formation and Type II supernovae.In the models where the supernova heating rate was very high in the past, the gas initially forms a transonic wind.As the rate of supernovae declines, these models may undergo a period of subsonic inflation.The heating of the gas is insufficient to unbind it from the galaxy,and most of the injected energy goes into increasing the pressure in the gas and causing it to inflate slowly.Finally, once the cooling time becomes shorter than the age of the galaxy,a steady-state coolingflow forms.In this phase,the X-ray luminosity of the galaxy is essentially equal to the total rate of heating of the gas.In§3.3,we noted that the cooling time is less than the age over essentially the entire observed regions of hot gas in the brighter X-ray ellipticals.Thus,these galaxies are likely to be undergoing steady-state cooling at present.5.Steady-State CoolingOne-dimensional,steady-state,coolingflow models for the gas in ellipticals have been calculated by Thomas(1986),Sarazin&White(1987),Vedder et al.(1988), Sarazin&Ashe(1989),and others.In these models,the rate of heat input associated with the injection of gas by stellar mass loss equal the rate of emission of the gas as it cools.Thus,the X-ray luminosity for steady cooling is given approximately byL X≈ 3kT injX-ray Emission7When combined with equations1and4,this leads to L X∝L1.5−2B ,consistentwith the observed relation.In Figure1,the predicted L X–L B relationship is shown for detailed coolingflow models from Sarazin&Ashe(1989).Steady cooling models give a reasonably goodfit to the radial X-ray surface brightness profiles of ellipticals.There is a simple argument which explains this (Sarazin1986).In steady cooling models,the rate of heating of the gas equals the rate of cooling through the emission of X-rays(equation4).If this applies locally as well as globally,then the emissivity of the gas equals the local heating rate per unit volume.If one integrates the X-ray emissivity along a line of sight through the galaxy,this givesI X(r)≈3µm pT inj ˙M∗Λ I B(r),(5)where the averages are along the line-of-sight at r.The factor ΛX/Λ is a bolometric correction for the fraction of the gas emission is the X-ray band,which is nearly unity.If the velocity dispersion of the galaxy is relatively constant,the T inj ,then equations1and5imply that I X∝I B,approximately as is observed. Also,if one adopts a Hubble law for the optical surface brightness of ellipticals, then this gives I X∝r−2Figure3.The radial variation of the temperature in NGC4472from the ROSAT PSPC X-ray spectrum(Irwin&Sarazin1996).The curve is afit to the data.Finally,the steady cooling models are consistent with the temperatures and temperature variations observed in the brighter X-ray ellipticals.The steady cooling models generally predict that the gas temperatures are relatively con-stant in the outer regions,and decline toward the center.ROSAT and ASCA spectra show this pattern in all of the bright ellipticals of which I am aware.For example,Figure3shows the observed temperature profile of NGC4472(Irwin &Sarazin1996).8Sarazin6.X-ray Faint EllipticalsMost of the detailed X-ray properties of ellipticals discussed above are based on observations of the brightest ellipticals.However,it is clear from Figure1that there is a range of at least an order of magnitude in the X-ray luminosities of ellipticals with the same optical luminosity.Why is there such a large dispersion in X-ray luminosities?What is the origin of the detected X-ray emission of the fainter ellipticals?Is it thermal emission by interstellar gas as in the brighter ellipticals?There have been a number of suggestions as to the origin in the dispersion in the X-ray luminosities of ellipticals.D’Ercole et al.(1989)suggested that the faint X-ray ellipticals are at an earlier evolutionary stage and undergoing subsonic inflation(§4).However,the specific version of this hypothesis which they advocated required a large supernova heating rate,which may be ruled out by the X-ray spectra of ellipticals and other X-ray data(§§3.2,7).White&Sarazin(1991)suggested that the X-ray faint ellipticals were mainly located in denser regions,and that the gas in these galaxies had been removed by ram pressure stripping.While there are clear cases of this occur-ring(e.g.,M86in the Virgo cluster),the statistical anticorrelation of X-ray luminosity with local density is not very well established(Eskridge et al.1995).What is the nature of the X-ray emission in the X-ray faint ellipticals?It might be due to residual diffuse hot ISM,in which case it would have a soft X-ray spectrum,or due to low mass X-ray binaries[LMXRBs],with a hard X-ray spectrum.In Figure1,the shaded area shows the predicted X-ray luminosities of due to LMXRBs based on a number of different extrapolations from nearby galaxies.Because the stellar populations of bright ellipticals appear to be fairly homogeneous,one expects the L X contribution of LMXRBs to scale with the optical luminosity L B.ASCA X-ray spectra of essentially all ellipticals show the presence of a very hard spectral component which is approximately proportional to the optical lu-minosity of the galaxy(Matsushita et al.1994;Matsumoto et al.1996).This hard spectral component is consistent in spectral shape andflux with that ex-pected from LMXRBs.So,it is likely that binary stars are an important source of X-ray emission in X-ray faint ellipticals.However,ROSAT and ASCA spectra also show evidence for a very soft X-ray spectral component in X-ray faint ellipticals(Fabbiano et al.1994).This component might be due to diffuse ISM,to stellar corona,or to some unknown cause,although there is some evidence favoring an interstellar origin(Davis& White1996).Thus,it seems likely that the X-ray emission of X-ray faint ellipticals is due to several mechanisms,including LMXRBs and residual hot ISM.X-ray Emission97.Abundances in the X-ray GasAt the temperatures found in elliptical galaxies,much of the X-ray emission is line emission due to heavy elements.Thus,X-ray spectra can be used to determine the abundances in the gas,particularly the abundance of iron.It was expected that the gaseous abundances would be moderately to extremely high, depending on the supernova rate.The heavy element abundances in stars in the central regions of elliptical galaxies are rather high,and Type Ia supernovae may further enhance the abundances.The expected average gaseous iron abundance is then(Fe/H)gas+1.6 M F e0.075 ,(6)(Fe/H)⊙where(Fe/H)∗is the iron abundance in the normal stellar ejecta,and each Type Ia supernova produces a mass M F e of iron.The supernova rate used in equation 6is at the low end of the suggested rates,so the iron abundances in ellipticals were expected to be(Fe/H)gas∼>3(Fe/H)⊙,and could reach ten times solar if higher supernova rates apply.The expected gaseous abundances in ellipticals are also sensitive to stellar abundance gradients and the dynamical state of the gas.The stellar abundance gradients observed in elliptical galaxies will produce gradients in the interstellar abundances,particularly for elements which are not provided mainly by Type Ia supernovae.If the gas in involved in an outflow(e.g.,D’Ercole et al.1989),the heavy elements at any radius will have come from stars interior to that radius, and this will increase the abundances.Conversely,if the gas is involved in an inflow as in a coolingflow,all of the heavy elements come from the outer regions of the galaxy,and the abundances are depressed.X-ray spectra from ROSAT and particularly ASCA show that the interstel-lar abundances of iron are quite low,(Fe/H)gas≈0.1−0.5(Fe/H)⊙(Awaki et al. 1994;Loewenstein et al.1994;Mushotzky et al.1994;Matsumoto et al.1996). In a number of cases,abundance gradients are seen.Such a low iron abundance requires that the rate of Type Ia supernovae mass injection must be very low (equation6).The low abundances and the presence of abundance gradients sug-gests that stellar abundances gradients and inflow affect the spectra.However, the observed abundances are so low that external inflow of low abundance gas or incomplete mixing of stellar ejecta into the hot gas may be required.It would be very helpful in this regard if accurate stellar iron abundance gradients could be determined for many of the X-ray bright ellipticals.ReferencesAwaki,H.,Koyama,K.,Kunieda,H.,Takano,S.,Tawara,Y.,&Ohashi,T.1991,ApJ,366,88Awaki,H.,et al.,1994,PASJ,46,L65Canizares,C.R.,Fabbiano,G.,&Trinchieri,G.1987,ApJ,312,503David,L.P.,Forman,W.,&Jones,C.1990,ApJ,359,2910SarazinDavis,D.S.,&White,R.E.,III,1996,preprintD’Ercole,A.,Renzini,A.,Ciotti,L.,&Pellegrini,S.1989,ApJ,341,L9 Eskridge,P.,Fabbiano,G.,&Kim,D.-W.1995,ApJS,97,141Evans,R.,van den Bergh,S.,&McClure,R.1989,ApJ,345,752 Fabbiano,G.,Gioia,I.,&Trinchieri,G.1989,ApJ,347,127Fabbiano,G.,Kim,D.-W.,&Trinchieri,G.1992,ApJS,80,531Fabbiano,G.,Kim,D.-W.,&Trinchieri,G.1994,ApJ,429,94Faber,S.M.,&Gallagher,J.S.1976,ApJ,204,365Forman,W.,Jones,C.,&Tucker,W.1985,ApJ,293,102Irwin,J.A.,&Sarazin,C.L.1996,ApJ,in pressLoewenstein,M.,&Mathews,W.G.1987,ApJ,319,614Loewenstein,M.,Mushotzky,R.,Tamura,T.,Ikebe,Y.,Makishima,K.,Mat-sushita,K.,Awaki,H.,&Serlemitsos,P.1994,ApJ,436,L75 Matsumoto,H.,Koyama,K.,Awaki,H.,Tsuru,T.,Loewenstein,M.,&Mat-sushita,K.1996,preprintMatsushita,K.,et al.,1994,ApJ,436,L41Mushotzky,R.,Loewenstein,M.,Awaki,H.,Makishima,K.,Matsushita,K.,& Matsumoto,H.1994,ApJ,436,L79Nulsen,P.E.,Stewart,G.C.,&Fabian,A.C.1984,MNRAS,208,185 Renzini,A.,&Buzzoni,A.1986,in Spectral Evolution in Galaxies,ed.C.Chiosi and A.Renzini(Dordrecht:Reidel),195Sarazin,C.L.1986.in Gaseous Halos around Galaxies,ed.J.Bregman&F.Lockman(Greenbank:NRAO),223Sarazin,C.L.1990,in The Interstellar Medium in Galaxies,ed.H.A.Thronson, Jr.,&J.M.Shull(Dordrecht:Kluwer),201Sarazin,C.L.,&Ashe,G.A.1989,ApJ,345,22Sarazin,C.L.,&White,R.E.,III,1987,ApJ,320,32Tammann,G.A.1982,in Supernovae:A Survey of Current Research,ed.by M.J.Rees&R.J.Stoneham(Dordrecht:Reidel),371Thomas,P.A.1986,MNRAS,220,949Trinchieri,G.,&Fabbiano,G.1985,ApJ,296,447Trinchieri,G.,Fabbiano,G.,&Canizares,C.R.1986,ApJ,310,637 Vedder,P.W.,Trester,J.J.,&Canizares,C.R.1988,ApJ,332,725White,R.E.,III,&Sarazin,C.L.1991,ApJ,367,476The work was supported by NASA Astrophysical Theory Program grant NAG 5-3057,NASA ASCA grant NAG5-2526,and NASA ROSAT grant5-3308.。
Spectral and temporal properties of X-ray emission from the ultra-luminous source X-9 in M8

We have analysed the spectra and the variability of individual X-ray sources in the M-81 field using data from the available ROSAations of this nearby spiral galaxy.
2 The data
We analysed the available archival data from ROSAT PSPC (12 observation, with 8 pointed on M 81 nucleus for a total exposure time of 146 ksec) and HRI (7 observations pointed on M 81 nucleus for a total exposure time of 135 ksec). We also used a SAX observation pointed on M 81 and one ASCA observation pointed on X-9.
Figure 3: Radial profiles of the calibration source HZ43 (stars), compared to X9 profile (diamonds). Left panel: external sector. Right panel: internal sector. Data points are normalized to the total number of counts within 2 arcmin. Extraction annuli are 2 arcsec wide (1 detector pixel = 0.5 arcsec)
Tests for supernova explosion models from light curves to X-ray emission of supernova remna

a r X i v :a s t r o -p h /0309146v 1 4 S e p 20032Elena Sorokina and Sergey BlinnikovTable1.Parameters of SN Ia modelsM WD a 1.38611.37750.86780.6500 1.4M56Ni a0.630.600.470.050.42E51b 1.23 1.20 1.150.560.46Tests for supernova explosion models3 Infig.1we compare the light curves inγ–rays produced by different models.We have chosen W7as a representative case of Chandrasekhar-mass models.The light curves inγ–rays are more or less similar for all of them.The luminosity of Sub-Chandrasekhar-mass MR model is lower due to smaller amount of56Ni,and it rises slower since it is less energetic,soγ–photons are locked inside the ejecta for longer time. One can see even smaller number ofγ–photons during thefirst weeks after the explosion in the model similar to MR but less mixed,with56Ni residing mostly near the center.The maximum luminosity inγis still the same as in original MR,since we preserve the total amount of56Ni.Therefore,the light curve inγ–rays during thefirst months can be divided into two epochs,that represent different physical parameters of the explosion:thefirst40–60days and the following evolution. From the observations during thefirst period one can judge on the combination of explosion energy and the distribution of radioactive stuffover the ejecta,while the second period tells us mostly about total production of radioactive elements at the explosion.Since currently the observations can only provide us withfluxes integrated over several days(or even weeks),it seems expedient to make two different sets of observations:during thefirst two months after the explosion,and after that,in order to distinguish between different parameters of explosion,and to test explosion models.We have described our modelling of bolometric and UBVRI light curves and calculations of X-ray emission of young SNRs in the previous work.For details one can see e.g.[7,8,9].We just mention here that a bit less energetic and more mixed models,like MR,seem to us more preferable both for SN light curve and for SNR X-ray emission.At the latter point,our results seem to contradict the conclusions by[10],that iron lines from well mixed models at the SNR stage are too strong,while less mixed models give a better agreement with observations of Tycho.Still we trust in our results more,since we take into account the ionization energy in the equation of state,which is comparable to the thermal energy.Electron thermal conductivity is also included into our calculations,and it smoothes very much the temperature profile between forward and reverse shocks.Both these effects are neglected in the code by[10],though they are able to change the emitted spectrum appreciably.The code by[10]takes into account possible difference between electron and ion temperature using the standard Coulomb collision equation,while we treat this effect parametrically.This can also lead to differences in our results.The work on improving physics and making our code more self-consistent is in progress.3.2SN1993JIn order to pay attention to the SN entitling this conference,we have recalculated its light curve.We use the same model13C from[11]which was investigated a few years ago in[12].It is one of the best models for SN1993J,and we just wanted to check if the new version of our code STELLA,with improved and renovated physics,still produces the light curve thatfits observations well.The results of previous and current calculations are compared infig.2.The main improvement in the code is a new approach for expansion opacities[13].They become much more complicated,and,most probably,the bumps on the new light curves are the results of this improvement.But sometimes(for instance,in the U band)these bumps seem tofit the observations even better than it was in the old version.R and I bands are calculated with STELLA for thefirst time,and they look perfect.There is a discussion in the literature on the estimates of the reddening to SN1993J([14]and references therein).In the plots infig.2we assume E(B−V)=0.08.With larger value,the observational curves become higher than the modelled ones.So the new version of STELLA confirms that the model13C corresponds to the explosion of SN1993J very well,but in the case of low reddening.Acknowledgements.ES is grateful to the organizers of the meeting for their warm hospitality and support. The work is supported by RFBR through grants02–02–16500,03–02–06770,and03–02–26598.References1.K.Nomoto,F.–K.Thielemann,K.Yokoi:ApJ,286,644(1984)2.S.E.Woosley,T.A.Weaver:Massive Stars,Supernovae,and Nucleosynthesis.In:Supernovae,ed by R.Blud-man et al(ELSEVIER Sci.Pub.,Amsterdam1994)p63–1543. E.Livne,D.Arnett:ApJ452,62(1995)4.P.Ruiz-Lapuente et al.:Nature365,728(1993)5.M.Reinecke,W.Hillebrandt,J.C.Niemeyer:A&A386,936(2002)6.R.Georgii,S.Pl¨u schke,R.Diehl et al:A&A394,517(2002)4Elena Sorokina and Sergey BlinnikovFig.2.Bolometric and UBVRI light curves for SN1993J.Top left panel shows the best model13C8from[12](solid line–calculations with STELLA,dashes–with EDDINGTON),top right and bottom panels:our recalculation of the same model with a new version of STELLA.Observational data are taken from[14]and shown by crosses. The value of reddening is taken to be E(B−V)=0.08.7.S.Blinnikov,E.Sorokina:Type Ia Supernova models:latest developments.In:Hunting the cosmologicalparameters,ed by D.Barbossa et al(KLUWER2003)(astro–ph/0212530)8.S.Blinnikov,E.Sorokina:A&A356,L30(2000)9. D.I.Kosenko,E.I.Sorokina,S.I.Blinnikov,P.Lundqvist:X-ray emission of young SN Ia remnants as a probefor an explosion model.In:Proc.of34th Scientific Assembly of COSPAR,(ASR2003)(astro-ph/0212188)10. C.Badenes,E.Bravo,K.J.Borkowski,I.Dom´ınguez:ApJ593358(2003)11.S.E.Woosley,R.G.Eastman,T.A.Weaver,P.A.Pinto:ApJ429,300(1994)12.S.I.Blinnikov,R.Eastman,O.S.Bartunov et al:ApJ496,454(1998)13. E.I.Sorokina,S.I.Blinnikov:Energy exchange inside SN ejecta and light curves of SNe Ia.In:NuclearAstrophysics,MPA/P13,ed by W.Hillebrandt,E.M¨u ller(Garching2002)pp57–6214.M.W.Richmond,R.R.Treffers,A.V.Filippenko et al:Astron.J.107,1022(1994)。
VW_01014 大众图纸图框及字体规范
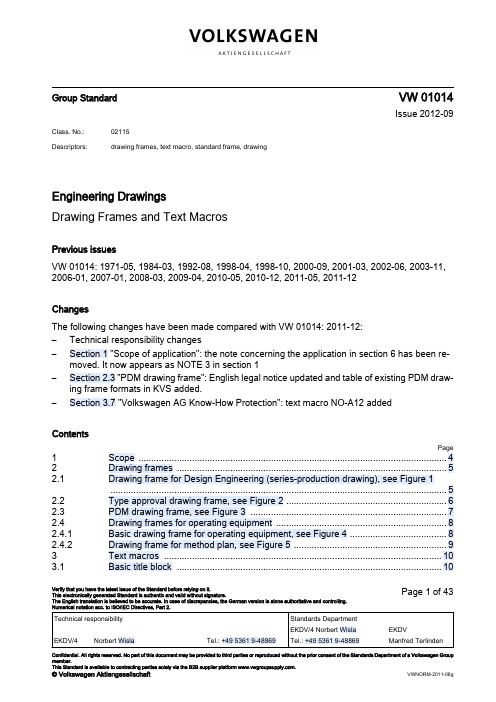
Engineering DrawingsDrawing Frames and Text MacrosPrevious issuesVW 01014: 1971-05, 1984-03, 1992-08, 1998-04, 1998-10, 2000-09, 2001-03, 2002-06, 2003-11,2006-01, 2007-01, 2008-03, 2009-04, 2010-05, 2010-12, 2011-05, 2011-12ChangesThe following changes have been made compared with VW 01014: 2011-12:–Technical responsibility changes–Section 1 "Scope of application": the note concerning the application in section 6 has been re‐moved. It now appears as NOTE 3 in section 1–Section 2.3 "PDM drawing frame": English legal notice updated and table of existing PDM draw‐ing frame formats in KVS added.–Section 3.7 "Volkswagen AG Know-How Protection": text macro NO-A12 added ContentsPageScope .........................................................................................................................4Drawing frames ..........................................................................................................5Drawing frame for Design Engineering (series-production drawing), see Figure 1....................................................................................................................................5Type approval drawing frame, see Figure 2 ...............................................................6PDM drawing frame, see Figure 3 .............................................................................7Drawing frames for operating equipment ...................................................................8Basic drawing frame for operating equipment, see Figure 4 ......................................8Drawing frame for method plan, see Figure 5 ............................................................9Text macros .............................................................................................................10Basic title block .. (10)122.12.22.32.42.4.12.4.233.1Group StandardVW 01014Issue 2012-09Class. No.:02115Descriptors:drawing frames, text macro, standard frame, drawingVerify that you have the latest issue of the Standard before relying on it.This electronically generated Standard is authentic and valid without signature.The English translation is believed to be accurate. In case of discrepancies, the German version is alone authoritative and controlling.Page 1 of 43Confidential. All rights reserved. No part of this document may be provided to third parties or reproduced without the prior consent of the Standards Department of a Volkswagen Group member.This Standard is available to contracting parties solely via the B2B supplier platform .© Volkswagen AktiengesellschaftVWNORM-2011-08gTitle blocks for drawings with restrictions on use .....................................................11Title block for layout drawings (ENT) > A0 ...............................................................12Symbol for European projection method ..................................................................13Change block for formats > A0 .................................................................................13Tolerancing principle as per VW 01054 ...................................................................13Volkswagen Group know-how protection .................................................................13Drawing field ............................................................................................................14Lower left corner of drawing for formats > A0 ..........................................................14Left drawing edge for formats > A0 ..........................................................................14Explanation of parenthesized dimensions for formats > A0 (lower left corner ofdrawing field) ............................................................................................................14References for formats > A0 ....................................................................................15Migration from CATIA V4 to CATIA V5 ....................................................................15Parts marking ...........................................................................................................15Part number assignment drawn / symmetrically opposite ........................................15Note on utilization of scrap material .........................................................................16NO-F1 Drawings with multiple sheets ......................................................................16Repeating and unchanging notes, mostly on body components ..............................16Drawing only for the company stated .......................................................................16Note on parts which are subject to build sample approval (BMG) ...........................17Notes on testing as per Technical Supply Specifications (TL) .................................17Note on type approval ..............................................................................................17Note on undimensioned design models in the data record ......................................17Note on open-air weathering ....................................................................................17Note on model approval ...........................................................................................17Note on master model ..............................................................................................18Note on second original, font size 7 mm ..................................................................18Note on second original, font size 3,5 mm ...............................................................18Note on heavy-duty component ...............................................................................18Note on mandatory type approval ............................................................................19Note on avoidance of hazardous substances ..........................................................19Note on other relevant drawings ..............................................................................19Note on undimensioned bend and trim radii ............................................................19Note on simplified representation .............................................................................19Note on flawless condition of surfaces .....................................................................19Note on material for form tool in grain area ..............................................................20Table for RPS ...........................................................................................................20Note on emission behavior .......................................................................................20Note on length dimensions to be measured up to relevant functional datum plane ..................................................................................................................................20Note on related tolerances for nominal dimension ranges up to relevant functional datum plane .............................................................................................................21Note on tolerances of surfaces as compared to the data record and defined RPS..................................................................................................................................21Note on tolerances of marked surfaces as compared to the data record anddefined RPS .............................................................................................................21Note on tolerances of marked and limited surfaces as compared to the datarecord and defined RPS ...........................................................................................21Note on tolerances of marked edges as compared to the data record and defined RPS ..........................................................................................................................21Note on alternative materials and surface protection types .....................................22Note on color and grain .. (22)3.23.33.43.53.63.744.14.24.34.44.54.64.74.84.94.104.114.124.134.144.154.164.174.184.194.204.214.224.234.244.254.264.274.284.294.304.314.324.334.344.354.364.374.38Page 2VW 01014: 2012-09Note on temperature resistance ...............................................................................22Note on color consistency ........................................................................................22Note on lightfastness ................................................................................................22Note on fixing, clamping and contact surface ..........................................................23Note on related finished part drawing ......................................................................23Note on material specifications, complete ................................................................23Note on material specifications, subdivided .............................................................24Note on optional welding technology .......................................................................24Note on flammability features ...................................................................................24Note on table containing gear tooth data .................................................................25Note on weight indication .........................................................................................25Note on amine emission of foam parts .....................................................................25Note on cleanliness requirements for engine components ......................................25Countersinks for internal threads .............................................................................26Testing of rolled bushings ........................................................................................26Table for limit dimensions ........................................................................................26Detail drawing for radius under screw head, mostly for standard part drawings (27)Test specification for disk wheels .............................................................................27Test specification for brake drums ...........................................................................28General tolerances for castings ...............................................................................28General tolerances for forgings ................................................................................29Coordinate dimensioning for tubes and bars ...........................................................30Bill of materials for layout drawings (ENT) ...............................................................30Distribution list for layout drawings (ENT) ................................................................31Text macros for operating equipment ......................................................................31Title block for individual part .....................................................................................31Note on pass direction, left .......................................................................................32Note on pass direction, right ....................................................................................32Title block for operating equipment label .................................................................32General tolerances for nominal dimensions without tolerance specification ............32Note on simplified drawing specifications on surface roughnesses .........................33Permissible deviations for nominal sizes without tolerance specification onweldments ................................................................................................................33Permissible deviations for nominal dimensions without tolerance specificationson flame-cut parts ....................................................................................................33Note on parts used ...................................................................................................34Note on rolled flame-cutting template plots ..............................................................34Note on "Add ½ kerf" ................................................................................................34Note on "designed" and "symmetrical opposite" ......................................................34Text macros for the "3D drawingless process" (3DZP – German abbreviation) ......35VW copyright ............................................................................................................35Note on restriction on use ........................................................................................35Note on type approval documentation and type approval number ...........................35Draft number ............................................................................................................36Note on engineering project number ........................................................................36Note on safety documentation .................................................................................36Recycling requirements as per VW 91102 ...............................................................36All dimensions apply to the finished part including surface protection .....................36Surface roughness as per VW 13705 and VDA 2005 ..............................................36Surface roughness as per VW 13705 and VDA 2005 (reference without symbol) (37)4.394.404.414.424.434.444.454.464.474.484.494.504.514.524.534.544.554.564.574.584.594.604.614.6255.15.25.35.45.55.65.75.85.95.105.115.1266.16.26.36.46.56.66.76.86.96.9.1Page 3VW 01014: 2012-09Surface roughness as per VW 13705 and VDA 2005 (reference with symbol) .......37Surface roughness as per VW 13705 and VDA 2005 (reference with symbol,collective specification 1) .........................................................................................38Surface roughness as per VW 13705 and VDA 2005 (reference with symbol,collective specification 2) .........................................................................................39Workpiece edges as per VW 01088 .........................................................................39Workpiece edges as per VW 01088 (reference without symbol) .............................40Workpiece edges as per VW 01088 (reference with symbol) ..................................40Workpiece edges as per VW 01088 (reference with symbol, collectivespecification 1) .........................................................................................................41Workpiece edges as per VW 01088 (reference with symbol, collectivespecification 2) .........................................................................................................42Applicable documents ..............................................................................................426.9.26.9.36.9.46.106.10.16.10.26.10.36.10.47ScopeThis standard applies to the computer-aided graphical representation and presentation of drawing templates, standard frames and text macros for drawings within the Volkswagen Group.NOTE 1 The standardized text macros are subject to drawing standard regulations and are centrally managed by the "Virtual Systems and Standardization" department.NOTE 2 All drawing frames and text macros shown here are available in the appropriate standard system environment of the CAD systems CATIA and Creo Elements/Pro (formerly PRO/E). The PDM drawing frames are also available as IsoDraw and Excel templates in the KVS, and also as Catia V5templates.NOTE 3 The text macros shown in section 6 are for the drawingless process only. The creator and the user of the data must agree whether their process chain allows for the use of documents as per the 3DZP method, and whether this is permissible.1Page 4VW 01014: 2012-09Drawing framesDrawing frame for Design Engineering (series-production drawing), see Figure 1Figure 1 – Drawing frame for Design Engineering (series-production drawing)2 2.1Page 5VW 01014: 2012-09Type approval drawing frame, see Figure 2Figure 2 – Type approval drawing frame2.2 Page 6VW 01014: 2012-09PDM drawing frame, see Figure 3Figure 3 – PDM drawing frame2.3 Page 7VW 01014: 2012-09Drawing frames for operating equipmentBasic drawing frame for operating equipment, see Figure 4Figure 4 – Basic drawing frame for operating equipment2.4 2.4.1Page 8VW 01014: 2012-09Drawing frame for method plan, see Figure 5Figure 5 – Drawing frame for method plan2.4.2 Page 9VW 01014: 2012-09Text macrosBasic title blockFigure 6 – Code no: NO-A1Basic title block for formats > A03 3.1Page 10VW 01014: 2012-09Title blocks for drawings with restrictions on useFigure 7 – Code no: NO-A7 A3The title block may only be used if supplier original drawings are used as modified finished part drawings.Notes on the usage of these title blocks see VW 01058.3.2Title block for layout drawings (ENT) > A0Figure 8 – Code no: NO-A3ENT = Draft3.3Symbol for European projection methodFigure 9 – Code no: NO-A5Change block for formats > A0Figure 10 – Code no: NO-A6Tolerancing principle as per VW 01054Figure 11 – Code no: NO-A11Volkswagen Group know-how protectionFigure 12 – Code no: NO-A123.4 3.5 3.6 3.7Drawing fieldLower left corner of drawing for formats > A0Figure 13 – Code no: NO-B1Left drawing edge for formats > A0Figure 14 – Code no: NO-B3Explanation of parenthesized dimensions for formats > A0 (lower left corner of drawingfield)Figure 15 – Code no: NO-B644.1 4.2 4.3References for formats > A0Figure 16 – Code no: NO-B7Migration from CATIA V4 to CATIA V5Figure 17 – Code no: NO-B8Parts markingFigure 18 – Code no: NO-E2Part number assignment drawn / symmetrically oppositeFigure 19 – Code no: NO-E54.4 4.5 4.6 4.7Note on utilization of scrap materialFigure 20 – Code no.:NO-F1 Drawings with multiple sheetsFigure 21 – Code no: NO-F2Repeating and unchanging notes, mostly on body componentsFigure 22 – Code no: NO-F3Drawing only for the company statedFigure 23 – Code no: NO-F4 (do not use for new designs!)4.8 4.9 4.10 4.11Note on parts which are subject to build sample approval (BMG)Figure 24 – Code no: NO-F5Notes on testing as per Technical Supply Specifications (TL)Figure 25 – Code no: NO-F6Note on type approvalFigure 26 – Code no: NO-F7Note on undimensioned design models in the data recordFigure 27 – Code no: NO-F8Note on open-air weatheringFigure 28 – Code no: NO-F9Note on model approvalFigure 29 – Code no: NO-F104.12 4.13 4.14 4.15 4.16 4.17Note on master modelFigure 30 – Code no: NO-F11Note on second original, font size 7 mmFigure 31 – Code no: NO-F12Note on second original, font size 3,5 mmFigure 32 – Code no: NO-F13Note on heavy-duty componentFigure 33 – Code no: NO-F144.18 4.19 4.20 4.21Note on mandatory type approvalFigure 34 – Code no: NO-F15Note on avoidance of hazardous substancesFigure 35 – Code no: NO-F16Note on other relevant drawingsFigure 36 – Code no: NO-F17Note on undimensioned bend and trim radiiFigure 37 – Code no: NO-F18Note on simplified representationFigure 38 – Code no: NO-F19Note on flawless condition of surfacesFigure 39 – Code no: NO-F204.22 4.23 4.24 4.25 4.26 4.27Note on material for form tool in grain areaFigure 40 – Code no: NO-F22Table for RPSFigure 41 – Code no: NO-F23Note on emission behaviorFigure 42 – Code no: NO-F24Note on length dimensions to be measured up to relevant functional datum planeFigure 43 – Code no: NO-F254.28 4.29 4.30 4.31Note on related tolerances for nominal dimension ranges up to relevant functional datumplaneFigure 44 – Code no: NO-F26Note on tolerances of surfaces as compared to the data record and defined RPSFigure 45 – Code no: NO-F27Note on tolerances of marked surfaces as compared to the data record and defined RPSFigure 46 – Code no: NO-F28Note on tolerances of marked and limited surfaces as compared to the data record anddefined RPSFigure 47 – Code no: NO-F29Note on tolerances of marked edges as compared to the data record and defined RPSFigure 48 – Code no: NO-F304.32 4.33 4.34 4.35 4.36Note on alternative materials and surface protection typesFigure 49 – Code no: NO-F31Note on color and grainFigure 50 – Code no: NO-F32Note on temperature resistanceFigure 51 – Code no: NO-F33Note on color consistencyFigure 52 – Code no: NO-F35Note on lightfastnessFigure 53 – Code no: NO-F364.37 4.38 4.39 4.40 4.41Note on fixing, clamping and contact surfaceFigure 54 – Code no: NO-F37Note on related finished part drawingFigure 55 – Code no: NO-F38Note on material specifications, completeFigure 56 – Code no: NO-F394.42 4.43 4.44Note on material specifications, subdividedFigure 57 – Code no: NO-F40Note on optional welding technologyFigure 58 – Code no: NO-F41Note on flammability featuresFigure 59 – Code no: NO-F424.45 4.46 4.47Note on table containing gear tooth dataFigure 60 – Code no: NO-F43Note on weight indicationFigure 61 – Code no: NO-F44Note on amine emission of foam partsFigure 62 – Code no: NO-F45Note on cleanliness requirements for engine componentsFigure 63 – Code no: NO-F464.48 4.49 4.50 4.51Countersinks for internal threadsFigure 64 – Code no: NO-G0Testing of rolled bushingsFigure 65 – Code no: NO-G1Table for limit dimensionsFigure 66 – Code no: NO-G24.52 4.53 4.54Detail drawing for radius under screw head, mostly for standard part drawingsFigure 67 – Code no: NO-G4Test specification for disk wheelsFigure 68 – Code no: NO-G64.55 4.56Test specification for brake drumsFigure 69 – Code no: NO-G7General tolerances for castingsFigure 70 – Code no: NO-G84.57 4.58General tolerances for forgingsFigure 71 – Code no: NO-G94.59Coordinate dimensioning for tubes and barsFigure 72 – Code no: NO-G10Bill of materials for layout drawings (ENT)Figure 73 – Code no: NO-H14.60 4.61Distribution list for layout drawings (ENT)Figure 74 – Code no: NO-H2Text macros for operating equipmentTitle block for individual partFigure 75 – Code no: R001 individual part4.62 55.1Note on pass direction, leftFigure 76 – Code no: R002 pass direction, leftNote on pass direction, rightFigure 77 – Code no: R003 pass direction, rightTitle block for operating equipment labelFigure 78 – Code no: R004 operating equipment labelGeneral tolerances for nominal dimensions without tolerance specificationFigure 79 – Code no: R005 machining operation5.2 5.35.45.5Note on simplified drawing specifications on surface roughnessesFigure 80 – Code no: R006 surfacesPermissible deviations for nominal sizes without tolerance specification on weldmentsFigure 81 – Code no: R007 welded partsPermissible deviations for nominal dimensions without tolerance specifications on flame-cut partsFigure 82 – Code no: R008 flame-cut parts5.6 5.75.8Note on parts usedFigure 83 – Code no: R009 parts usedNote on rolled flame-cutting template plotsFigure 84 – Code no: R010 flame-cutting templateNote on "Add ½ kerf"Figure 85 – Code no: R011 kerfNote on "designed" and "symmetrical opposite"Figure 86 – Code no: R012 symmetrical opposite5.9 5.105.115.12Text macros for the "3D drawingless process" (3DZP – German abbreviation)The following text macros are not created in CAD systems, but only in the PDM system KVS.The design engineer must add the necessary parameters to the text macros.VW copyrightFigure 87 – Code no: NOZ-01Note on restriction on useLegend P01Company nameFigure 88 – Code no: NOZ-02Note on type approval documentation and type approval numberLegend P01Type approval doc. and type approval numberFigure 89 – Code no: NOZ-036 6.16.26.3Draft numberLegend P01Draft numberFigure 90 – Code no: NOZ-04Note on engineering project numberLegend P01Engineering project numberFigure 91 – Code no: NOZ-05Note on safety documentationLegend P01TLD number (technical guideline for documentation – German abbreviation)Figure 92 – Code no: NOZ-06Recycling requirements as per VW 91102Figure 93 – Code no: NOZ-07All dimensions apply to the finished part including surface protectionFigure 94 – Code no: NOZ-08Surface roughness as per VW 13705 and VDA 2005The design engineer must add the required parameters to the symbols shown here (e.g., Rz value).Two types of text macros (with and without graphical representation) have been defined. Variant NOZ-09 is a reference to Standard VW 13705, additional information possible, but restricted. Variants NOZ-09-01 a to f are reserved for the main surface roughness value. Due to system restrictions,identical symbols cannot be used more than once. For this reason, the symbols in section 6.9.3 and6.4 6.56.66.76.86.9section 6.9.4 must be used for cases of multiple use. If surface roughness values are added as a note, the text macros are placed beneath each other instead of beside each other. This deviating representation has been released for the 3DZP drawingless process.Surface roughness as per VW 13705 and VDA 2005 (reference without symbol)Figure 95 – Code no: NOZ-09Surface roughness as per VW 13705 and VDA 2005 (reference with symbol)Figure 96 – Code no: NOZ-09-01-aFigure 97 – Code no: NOZ-09-01-bFigure 98 – Code no.: NOZ-09-01-cLegend P01Machining allowance (numerical value in mm)P02Production processP03Surface parameter and numerical valueP04if applicable, additional requirement as per VDA 2005P05if applicable, additional requirement as per VDA 2005P06if applicable, second requirement on surface texture (surface parameter,numerical value)P07Specification of the surface groovesLegend P01Letter for simplified drawing specification. Method defined in section "simplified specifi‐cation" in VDA 2005Figure 99 – Code no: NOZ-09-01-d6.9.16.9.2Figure 100 – Code no: NOZ-09-01-e Figure 101 – Code no: NOZ-09-01-fSurface roughness as per VW 13705 and VDA 2005 (reference with symbol, collectivespecification 1)Figure 102 – Code no: NOZ-09-02-aFigure 103 – Code no: NOZ-09-02-bFigure 104 – Code no: NOZ-09-02-cLegend P01Machining allowance (numerical value in mm)P02Production processP03Surface parameter and numerical valueP04if applicable, additional requirement as per VDA 2005P05if applicable, additional requirement as per VDA 2005P06if applicable, second requirement onsurface texture (surface parameter,numerical value) P07Specifica‐tion of thesurface groovesCode no.:Legend P01Letter for simplified drawing specification. Method defined in section "simplified specifi‐cation" in VDA 2005Figure 105 – NOZ-09-02-dFigure 106 – Code no: NOZ-09-02-e Figure 107 – Code no: NOZ-09-02-f6.9.3。
x射线荧光光谱英文缩写

x射线荧光光谱英文缩写X-ray Fluorescence (XRF) Spectrometry: An Introduction and Applications.X-ray Fluorescence (XRF) spectrometry is a non-destructive analytical technique that utilizes the interaction of high-energy X-rays with matter to determine the elemental composition of materials. The technique is based on the fluorescence effect, where X-rays emitted from a source are absorbed by the sample, causing atoms within the sample to emit characteristic X-rays known as secondary or fluorescent X-rays. The emitted X-rays are then detected and measured to provide qualitative and quantitative information about the elements present in the sample.The fundamental principle of XRF spectrometry lies in the absorption and emission of X-rays by matter. When high-energy X-rays interact with a sample, they are absorbed by the atoms within the sample. This absorption process causes the inner-shell electrons of the atoms to be ejected,leaving vacancies in the electron shells. The outer-shell electrons then move down to fill these vacancies, releasing energy in the form of characteristic X-rays. The emitted X-rays have specific wavelengths or energies that are characteristic of the elements present in the sample.XRF spectrometers are typically divided into two categories based on the source of X-rays used: wavelength-dispersive XRF (WDXRF) and energy-dispersive XRF (EDXRF). In WDXRF spectrometers, a polychromatic X-ray source is used to excite the sample, and the emitted X-rays are then separated by a wavelength-dispersive monochromator into individual wavelengths. These wavelengths are then detected and measured using a suitable detector. In EDXRF spectrometers, a monochromatic X-ray source is used, and the emitted X-rays are detected and measured using an energy-dispersive detector that can resolve the X-rays based on their energy.XRF spectrometry has a wide range of applications in various industries and fields. In the metallurgical industry, XRF is commonly used for the determination ofelemental composition in metals, alloys, and ores. It is also used in the cement and glass industries to monitor the composition of raw materials and finished products. In environmental science, XRF spectrometry is employed for the analysis of soil, sediment, and water samples to assess pollution and contamination levels.In addition, XRF spectrometry plays a crucial role in the arts and antiquities industry. It can be used to authenticate artworks, such as paintings and sculptures, by analyzing the elemental composition of the pigments and materials used in their creation. Similarly, XRF can be used to identify and authenticate historical artifacts and jewelry by determining their elemental composition.The advantages of XRF spectrometry include its non-destructive nature, speed, and accuracy. Since the technique does not require any sample preparation or chemical reactions, it can be used on a wide variety of materials, including solids, liquids, and powders. Additionally, XRF spectrometers are relatively easy to operate and provide rapid results, making them suitable forboth laboratory and on-site applications.However, there are also some limitations to XRF spectrometry. One of the main limitations is that it is primarily suitable for the analysis of elements with atomic numbers greater than 11 (sodium). Lighter elements, such as hydrogen, helium, and lithium, cannot be detected using XRF spectrometry. Additionally, the technique is sensitive to surface conditions and can be affected by factors such as sample roughness, coating, and contamination.In conclusion, X-ray Fluorescence (XRF) spectrometry is a powerful analytical technique that provides rapid and accurate elemental composition analysis of materials. Its non-destructive nature, ease of operation, and wide range of applications make it a valuable tool in various industries and fields, including metallurgy, environmental science, arts and antiquities, and more. While there are some limitations to the technique, its overall advantages make it a preferred choice for elemental analysis in many scenarios.。
- 1、下载文档前请自行甄别文档内容的完整性,平台不提供额外的编辑、内容补充、找答案等附加服务。
- 2、"仅部分预览"的文档,不可在线预览部分如存在完整性等问题,可反馈申请退款(可完整预览的文档不适用该条件!)。
- 3、如文档侵犯您的权益,请联系客服反馈,我们会尽快为您处理(人工客服工作时间:9:00-18:30)。
a r X i v :a s t r o -p h /0205373v 1 22 M a y 2002Draft version February 1,2008Preprint typeset using L A T E X style emulateapj v.14/09/00X-RAY EMISSION FROM THE TYPE IC SUPERNOVA 1994I OBSERVED WITH CHANDRAStefan ImmlerAstronomy Department,University of Massachusetts,Amherst,MA 01003Andrew S.Wilson &Yuichi Terashima 1Astronomy Department,University of Maryland,College Park,MD 20742Draft version February 1,2008ABSTRACTWe present two high-resolution Chandra X-ray observations of supernova (SN)1994I which show,for the first time,that the interaction of the blast wave from a Type Ic SN with its surrounding circumstellar material (CSM)can give rise to soft X-ray emission.Given a 0.3–2keV band X-ray luminosity of L x ∼1×1037ergs s −1between six and seven years after the outburst of SN 1994I,and assumingthe X-ray emission arises from the shock-heated CSM,we derive a pre-SN mass-loss rate of ˙M∼1×10−5M ⊙yr −1(v w /10km s −1).Combining the results with earlier ROSAT observations,we construct the X-ray lightcurve of SN 1994I.A best-fit X-ray rate of decline of L x ∝t −s with index s ∼1and a CSM density profile of ρcsm ∝r −1.9±0.1are inferred,consistent with what is expected for a constant mass-loss rate and constant wind velocity profile for the SN progenitor (ρcsm ∝r −2).Subject headings:supernovae:individual (SN 1994I)—stars:mass loss —X-rays:individual (SN1994I)—X-rays:ISM1.introductionThe classification scheme for SNe is based on the pres-ence or absence of hydrogen lines in their optical spectra (Type II and Type I,respectively).While Type II/Ib SNe occur during the core collapse of a massive star,Type Ia SNe are believed to originate from the deflagration or deto-nation of an accreting white dwarf star in a binary system.The massive (>10M ⊙)progenitor stars for the controver-sial Type Ic SN subclass are thought to have lost their outer hydrogen and helium layers either through stellar winds or by mass transfer to a companion,leaving behind a stripped carbon and oxygen (C+O)star.Such a ‘naked’C+O star will later explode when its iron core collapses (e.g.Nomoto et al.1994).The interaction of the out-going SN shock wave with the ambient CSM,deposited either by a pre-SN stellar wind or non-conservative mass transfer to a companion,produces hot gas with character-istic temperatures in the range T ∼107–109K (Chevalier &Fransson 1994).Gas heated to such high temperatures produces radiation predominantly in the X-ray range.X-ray emission from this interaction is expected for all Type Ib/c and II SNe with substantial CSM established by the massive progenitors.Over the last 20years,searches for X-ray emitting SNe have been successful for only a rel-atively small number of Type II’s in the near aftermath (days to months)of the explosion (see Immler &Lewin 2002for a review article)2.By contrast,no Type Ia or Ib/c SN has ever been firmly detected in X-rays,apart from a 3.5σexcess in an XMM-Newton EPIC-PN image 4′′offset from the optical posi-tion of the Ic SN 2002ap (Rodriguez-Pascual et al.2002).Hard (2–10keV band)X-ray emission was recorded with BeppoSax from the position of the unusual SN 1998bw,which might be associated with a γ-ray burst event (GRB980425;Galama et al.1998,Pian et al.1998).Due to the large error box of the BeppoSax Wide Field Camera observation (between 3′and 8′,99%confidence limit)and the rather large probability of ∼60%that the source is a random chance coincidence,the association of the X-ray source with SN 1998bw and GRB 980425is still tentative (Galama et al.1998).Evidence for soft (0.1–2.4keV band)X-ray emission has been reported from the Type Ic SN 1994I,based on ROSAT HRI observations 82days after the outburst (Immler,Pietsch &Aschenbach 1998).However,the ROSAT observations,with a spatial resolution of ∼5′′(FWHM on-axis),were not conclusive since SN 1994I is lo-cated close to the X-ray bright nucleus of the host galaxy M51(distance ∼18′′)and is embedded in a high level of extended X-ray emission from hot gas and unresolved point-like X-ray sources in the bulge of M51.2.x-ray observations and analysisWe used the Chandra X-ray observatory to search for X-ray emission from SN 1994I in X-ray images taken between six (June 6,2000)and seven years (June 23,2001)after the explosion (March 31,1994;Chandler,Phillips &Ru-pen 1994).Our 14.9ks and 26.8ks Chandra observations were carried out with the S3chip of the Advanced CCD Imaging Spectrometer (ACIS-S)at the focal plane of the telescope and the nucleus of the host galaxy M51placed at the aim-point (Terashima &Wilson 2001).The superb spatial resolution (0.′′5FWHM on-axis),together with the high sensitivity of the instrument and the long combined exposure (41.7ks),allows us to separate point-like sources from the diffuse emission in the bulge of M51and to carry out a sensitive search for X-ray emission from SN 1994I.The co-added and adaptively smoothed Chandra image of the central region of M51is presented in Fig.1.1Institute of Space and Astronautical Science,3-1-1Yoshinodai,Sagamihara,Kanagawa 229-8510,Japan 2A complete list of X-ray SNe is available at /sne.html12Immler,Wilson &TerashimaAn X-ray source is detected at the position of SN 1994I in the merged Chandra data with a significance of ∼6σin the 0.3–2keV band using the ‘wave detect’algo-rithm implemented in the ciao data analysis package.The position of the X-ray source (R .A .,Dec .(2000)=13h 29m 54.s 17,+47◦11′30.′′2)is fully consistent with the ra-dio position of the SN (∼0.′′5offset;Rupen et al.1994).Astrometry of the M51nucleus shows a similarly small off-set between the (2–8keV band)X-ray and radio positions (∼0.′′3).Exposure corrected source counts were extracted within a radius of 3image pixels (90%encircled energy radius at 1keV)and corrected for the background taken in an annulus with inner and outer radii of 3.5and 9.5pixels,respectively (1pixel corresponds to 0.′′49).A spectral model has to be assumed to convert source counts into energy fluxes.We adopted an effective (0.3–2keV band)cooling function of Λ=3×10−23ergs cm 3s −1for an optically thin Raymond-Smith thermal plasma with a temperature of 107K (Raymond,Cox &Smith 1976).Although the temperature is unknown,this value is con-sistent with theoretical expectations (Chevalier &Frans-son 1994),previous X-ray observations of other SNe and the detection of SN 1994I in the soft (0.3–2keV)X-ray band where the peak of a 107K spectrum is located.The equivalent count rate to (unabsorbed)flux conversion fac-tors are then 4×10−11(ergs cm −2s −1)/(counts s −1)and 3×10−12(ergs cm −2s −1)/(counts s −1)for the ROSAT HRI and Chandra ACIS-S3,respectively,and a Galac-tic foreground column density of N H =1.3×1020cm −2(Dickey &Lockman 1990).The uncertainty of the conver-sion factors for optically thin thermal spectra with tem-peratures in the range 107–109K is ∼15%.Assuming a 0.86keV thermal bremsstrahlung spectrum (correspond-ing to a temperature of 107K)instead of a Raymond-Smith thermal plasma increases the conversion factor by ∼7%.The Chandra results for the two individual obser-vations of SN 1994I,together with previous ROSAT HRI results (Immler,Pietsch &Aschenbach 1998),are summa-rized in Table 1.3.discussionWe used the Chandra and ROSAT data to construct a combined X-ray lightcurve of SN 1994I,which is presented in Fig.2.A best-fit single power-law rate of decline off x ∝t −s with index s =0.9+0.1−0.2(solid line,Fig.2)is in-ferred for the measurements on days 82,2,271and 2,639.Assuming an initial exponential rise of the X-ray lumi-nosity after the outburst (at time t 0)of SN 1994I and a subsequent power-law decline with index s ,we also param-eterized the X-ray evolution as f x ∝(t −t 0)−s ×e −τwith τ∝(t −t 0)−β.This model has been successfully used to describe the time dependence of the radio emission of SNe (Weiler et al.1996).The external absorption of the emis-sion is represented by the e −τterm (‘optical depth’)and the time-dependence of the optical depth is parameterized by the exponent β.In the X-ray regime,the rise could represent either decreasing absorption by material along the line of sight to the hot gas or simply non-production of X-rays in the ROSAT band at early times.If we adopt this strictly heuristic description,we find s ∼1and β∼2(dashed line,Fig.2).In case the ROSAT detection on day 82corresponds to a pre-maximum measurement,a steeperindex of s ∼1.5is inferred (dotted line,Fig.2).However,given the large error range of the flux measurements,s and βare not very well constrained.While a t −1rate of decline has been observed for the Type IIb SN 1999em with Chandra (Pooley et al.2002),faster rates of decline have been reported for the Ic SN 1998bw (s =1.4),inferred from the tentative hard (2–10keV)band BeppoSax data (Pian et al.1999),the Ic radio SN 1990B (s =1.3)and the Ib radio SNe 1983N (s =1.6)and 1984L (s =1.5;Van Dyk et al.1993).By contrast,the long-term X-ray lightcurve of the IIb SN 1993J is best described by a slow rate of decline with s =0.27(Immler,Aschenbach &Wang 2001).The X-ray emitting material could be either shocked SN ejecta (reverse shock)or shocked CSM (forward shock;Chevalier &Fransson 1994).The lack of time-dependent X-ray spectroscopy precludes a distinction but,as we shall see,the X-ray lightcurve is consistent with the latter model with a constant pre-SN mass loss rate (ρcsm ∝r −2).Al-ternatively,the CSM might result from a non-conservative mass-transfer to the companion star,leading to the forma-tion of a flattened or disk-like H and He-rich shell (Nomoto et al.1994).In this case a CSM profile flatter than ρcsm ∝r −2is expected due to the non-spherically sym-metric geometry of the mass-transfer.In the stellar wind scenario the continuum equationrequires a mass-loss rate of ˙M=4πr 2ρw (r )×v w (r )through a sphere of radius r .After the SN shock plows through the CSM,its density is ρcsm =4ρw (Fransson,Lundqvist &Chevalier 1996).The X-ray luminosity of the shock-heated CSM is L x =Λ(T )d V n 2,where d V is the volume,n =ρcsm /m is the number density of the shocked CSM and m is the mean mass per particle (2.1×10−27kg for a H+He plasma).We thus obtainL x =4/(πm 2)Λ(T )×(˙M/vw )2×(v s t )−1.The observed X-ray rate of decline (t −1)is consistent with this descrip-tion if Λ(T ),˙M/vw and v s are constant.We can hence use the observed X-ray luminosity at time t after the out-burst to measure the ratio ˙M/vw assuming a constant shell expansion velocity v s .Given our observed X-ray luminosi-ties (Table 1)and assuming a shell expansion velocity of v s =16,500km s −1(Filippenko et al.1995)we derive amass-loss rate of ˙M∼1×10−5M ⊙yr −1(v w /10km s −1)consistent with all three detections and the ROSAT upper limit on day 1,368.It should be noted,however,that a single power-law model can only be reconciled with the data obtained on days 82–2,639,but not with the early ROSAT upper limit on day 52(see Fig.2).This is indicative that the above model might be incomplete for early epochs.The addi-tion of an exponential term describing the rise during the early phase of the emission leads to a fit that is in agree-ment with all measurements in case the ROSAT detection on day 82was a post-maximum measurement (s ∼1).By contrast,the model with a steeper rate of decline (s ∼1.5),which postulates that the early ROSAT detection corre-sponds to a pre-maximum measurement,is in conflict with a ρcsm ∝r −2profile.Table 1summarizes the CSM number density n for the different radii r =v s ×t corresponding to the dates of the observations.As was already claimed based on the early ROSAT data (Immler,Pietsch &Aschenbach 1998),theX-ray emission from the Type Ic SN1994I33σupper limit for the CSM density at r=2.0×1017cm (day1,368)is lower than that at1.2×1016cm(day82). Our Chandra results at3.2and3.8×1017cm(days2,271 and2,639,respectively)clearly support these early indi-cations for a decreasing CSM density profile(see Table1). The combined ROSAT and Chandra data of SN1994I give a best-fit profile ofρcsm∝r−1.9±0.1.The only SN for which a CSM density profile has been constructed from X-ray measurements is SN1993J(Type IIb with a progenitor of∼15M⊙),based on long-term monitoring with ROSAT between six days andfive years after the outburst(Immler,Aschenbach&Wang2001).A comparison between the CSM profiles of the two SNe is presented in Fig.3.Although the CSM density profile of SN1993J is significantlyflatter(ρcsm∝r−1.6)than that of SN1994I,the two SNe have a similar CSM number den-sity of∼106.5cm−3at a radius of r∼5×1015cm from the site of the explosion(cf.Fig.3).Given that both the X-ray rate of decline(L x∝t−1) and the CSM profile(ρcsm∝r−1.9±0.1)of SN1994I are not in conflict with what is expected for a constant stellar wind speed and a constant mass-loss rate of the progenitor (L x∝t−1,ρcsm∝r−2),it is likely that the stellar wind of the massive progenitor dominates the CSM.We thank Roger Chevalier for helpful discussion and the referee(Bernd Aschenbach)for his comments that led to significant improvement of the manuscript.This research was supported by NASA grants NAG5-8999to the Univer-sity of Massachusetts and NAG81755to the University of Maryland.Y.T.is supported by the Japan Society for the Promotion of Science Postdoctoral Fellowship for Young Scientists.REFERENCESChandler,C.J.,Phillips,J.A.,&Rupen,M.P.1994,IAU Circ.,No. 5978Chevalier,R.A.,&Fransson,C.1994,ApJ,420,268Dickey,J.M.,&Lockman,F.J.1990,ARA&A,28,215 Feldmeier,J.J.,Ciardullo,R.,&Jacoby,G.H.1997,ApJ,479,231 Filippenko,A.V.,et al.1995,ApJ,450,L11Fransson,C.,Lundqvist,P.,&Chevalier,R.A.1996,ApJ,461,993 Galama,T.J.,et al.1998,Nature,395,670Immler,S.,Pietsch,W.,&Aschenbach,B.1998,A&A,336,L1 Immler,S.,Aschenbach,B.,&Wang,Q.D.2001,ApJ,561,L107 Immler,S.,&Lewin,H.G.2002,in Supernovae and Gamma-Ray Bursts,ed.K.W.Weiler(Springer Publisher),astro-ph/0202231Nomoto,K.,et al.1994,Nature,371,227Pian,E.,et al.1999,A&AS,138,463Pooley,D.,et al.2002,ApJ in press,astro-ph/0103196 Raymond,J.C.,Cox,D.P.,&Smith,B.W.1976,ApJ,204,290 Rodriguez-Pascual,P.M.,et al.2002,IAU Circ.,No.7821 Rupen,M.P.,et al.1994,IAU Circ.,No.5963Terashima,Y.,&Wilson,A.S.2001,ApJ,560,139Van Dyk,S.,Sramek,R.A.,Weiler,K.W.,&Panagia,N.1993,ApJ, 419,162Weiler,K.W.,et al.1986,ApJ,301,790Table1X-ray Properties of SN1994IDay(a)Instrument Count Rate f x(b)L x(c)˙M(d)log n(e)(10−4cts s−1)(10−15)(1037)(10−5)(cm−3)(a)day after the outburst of SN1994I(March31,1994;Chandler,Phillips&Rupen1994)(b)0.3–2keV bandflux in units of10−15ergs cm−2s−1for a107K thermal plasma spectrum and a Galactic foreground column density of N H=1.3×1020cm−2(Dickey&Lockman1990)(c)0.3–2keV band luminosity in units of1037ergs s−1for an assumed distance of d=8.4Mpc (Feldmeier,Ciardullo&Jacoby1997)(d)pre-SN mass-loss rate in units of10−5M⊙yr−1(e)post-shock CSM number density4SN 1994Iband X-ray image of the central region of M51.The imagea plotted in logarithmic greyscale.The position of SN1994IofFig.2.—Soft(0.3–2keV)band X-ray lightcurve of SN1994I.The Chandra detections are marked byfilled boxes,open boxes indicate the early ROSAT HRI detection and3σupper limits.Error bars are±1σstatistical errors.Time is given in days after the outburst.The solid line represents a power-lawfit to the detections at days82,2,271and2,639.The dashed and dotted lines represent models including an e−τterm(see Section3).X-ray emission from the Type Ic SN1994I5Fig. 3.—Circumstellar matter density profile as a function of SN shell expansion radius.The solid line gives the best-fit CSM density profile ofρcsm∝r−1.9for the ROSAT(open boxes)and Chandra(filled boxes)measurements of SN1994I.The CSM density profile with ρcsm∝r−1.6based on ROSAT observations of SN1993J is drawn for comparison(dashed line and open triangles;Immler,Aschenbach& Wang2001).。