Asymmetric hydrogenation
一种双膦配体的合成
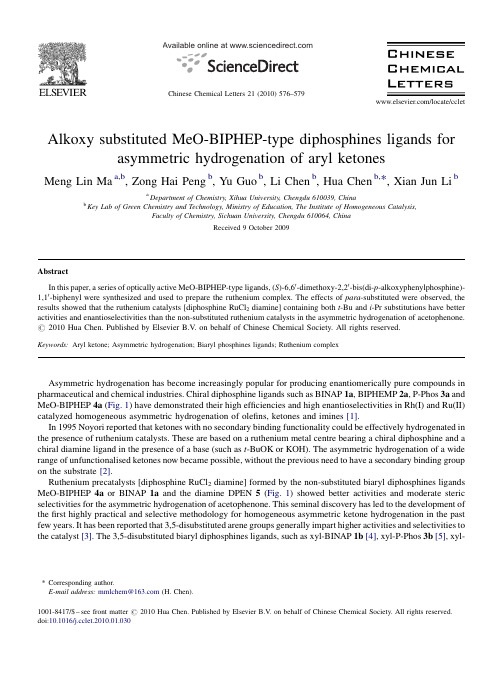
Alkoxy substituted MeO-BIPHEP-type diphosphines ligands forasymmetric hydrogenation of aryl ketonesMeng Lin Ma a ,b ,Zong Hai Peng b ,Yu Guo b ,Li Chen b ,Hua Chen b ,*,Xian Jun Li baDepartment of Chemistry,Xihua University,Chengdu 610039,ChinabKey Lab of Green Chemistry and Technology,Ministry of Education,The Institute of Homogeneous Catalysis,Faculty of Chemistry,Sichuan University,Chengdu 610064,ChinaReceived 9October 2009AbstractIn this paper,a series of optically active MeO-BIPHEP-type ligands,(S )-6,60-dimethoxy-2,20-bis(di-p -alkoxyphenylphosphine)-1,10-biphenyl were synthesized and used to prepare the ruthenium complex.The effects of para -substituted were observed,the results showed that the ruthenium catalysts [diphosphine RuCl 2diamine]containing both t -Bu and i -Pr substitutions have better activities and enantioselectivities than the non-substituted ruthenium catalysts in the asymmetric hydrogenation of acetophenone.#2010Hua Chen.Published by Elsevier B.V .on behalf of Chinese Chemical Society.All rights reserved.Keywords:Aryl ketone;Asymmetric hydrogenation;Biaryl phosphines ligands;Ruthenium complexAsymmetric hydrogenation has become increasingly popular for producing enantiomerically pure compounds in pharmaceutical and chemical industries.Chiral diphosphine ligands such as BINAP 1a ,BIPHEMP 2a ,P-Phos 3a and MeO-BIPHEP 4a (Fig.1)have demonstrated their high efficiencies and high enantioselectivities in Rh(I)and Ru(II)catalyzed homogeneous asymmetric hydrogenation of olefins,ketones and imines [1].In 1995Noyori reported that ketones with no secondary binding functionality could be effectively hydrogenated in the presence of ruthenium catalysts.These are based on a ruthenium metal centre bearing a chiral diphosphine and a chiral diamine ligand in the presence of a base (such as t -BuOK or KOH).The asymmetric hydrogenation of a wide range of unfunctionalised ketones now became possible,without the previous need to have a secondary binding group on the substrate [2].Ruthenium precatalysts [diphosphine RuCl 2diamine]formed by the non-substituted biaryl diphosphines ligands MeO-BIPHEP 4a or BINAP 1a and the diamine DPEN 5(Fig.1)showed better activities and moderate steric selectivities for the asymmetric hydrogenation of acetophenone.This seminal discovery has led to the development of the first highly practical and selective methodology for homogeneous asymmetric ketone hydrogenation in the past few years.It has been reported that 3,5-disubstituted arene groups generally impart higher activities and selectivities to the catalyst [3].The 3,5-disubstituted biaryl diphosphines ligands,such as xyl-BINAP 1b [4],xyl-P-Phos 3b [5],xyl-/locate/ccletAvailable online at Chinese Chemical Letters 21(2010)576–579*Corresponding author.E-mail address:mmlchem@ (H.Chen).1001-8417/$–see front matter #2010Hua Chen.Published by Elsevier B.V .on behalf of Chinese Chemical Society.All rights reserved.doi:10.1016/let.2010.01.030MeO-BIPHEP 4b [6]and xyl-TetraPHEMP 2b [7](Fig.1),were used in asymmetric hydrogenation of ketones and get good results,in which the steric influence of the 3,5-disubstituted was investigated.Due to the importance of the nature of substituents on the phosphorus atoms,we investigated the influence of newly designed MeO-BIPHEP-type atropisomeric diphosphines with para -alkoxy phenyl group at each of the phosphorus atom (Fig.2).The different structures of the alkoxy are expected to provide an opportunity to investigate the influence of the para -substituent in the asymmetric hydrogenation of ketones.In this paper,a series of chiral diphosphines 6,60-dimethoxy-2,20-bis(di-p -alkoxyphenylphosphine)-1,10-biphenyl were synthesized by an improvement way (Scheme 1)based on the Schmid method [8]and our improve way [9].In order to evaluate alkoxy substituted ligands,their corresponding ruthenium complexes were prepared according to literature methods [10](Scheme 2)and tested on the hydrogenation of acetophenone.Parallel experiments were performed to make direct comparison between our catalysts and reported ones.Basic reaction conditions were first optimized using acetophenone as substrate and p -methoxy-MeO-BIPHEP 4c phosphine ligand complex as a catalyst.M.L.Ma et al./Chinese Chemical Letters 21(2010)576–579577Fig.1.Ligands used for ketone hydrogenationcatalysts.Fig.2.Our biaryl diphosphineligands.Scheme 1.Reagents and conditions:(a)P(OEt)3,anhydrous NiCl 2,1408C,1h,87%yield or microwave,5min,94%yield,(b)(1)LDA in THF,À788C,(2)anhydrous FeCl 3in THF,À788C to rt.,16h,49%yield or (1)LTMP (BuLi +2,2,6,6-tetramethylpiperidine)in THF,À788C,(2)anhydrous FeCl 3in THF,À788C to rt.,16h,58%yield,(c)(1)(À)-DBTA in CH 2Cl 2/Et 2O,(2)10%NaOH/CH 2Cl 2,>99%ee,(d)SOCl 2,DMF,99%yield,(e)ArMgBr in THF,À788C to rt.,3h,95–68%yield or ArLi in THF,À788C to rt.,0.5h,46–35%yield,(f)HSiCl 3,Et 3N in toluene,reflux,98%yield,>99%ee.The catalytic activities of these ruthenium complexes in the hydrogenation of acetophenone were tested in i -Pr-OH,with a substrate/catalyst/base ratio (S/C/B)of 3000/1/100,at 508C and under 40bar of hydrogen (Table 1).The same ruthenium complexes were prepared with the MeO-BIPHEP in order to compare the activity and selectivity of ligands.As we have already observed with ligands 4c ,4d and 4e ,these alkoxy substituted almost have no influence either on the activity or on the selectivity (Table 1,entry 2,3and 4).Moreover some other para -substituent of the diphosphine ligands has the influence in the asymmetric hydrogenation of ketones.In our reaction conditions,the non-substituted MeO-BIPHEP ligands 4a provided 99%conversion to products and gave 81%ee at 208C (Table 1,entry 1).Different results were obtained because of their different chemical structures.The ligands substituted by para-t -Bu 4h and para-i -Pr 4f both gave good ee than non-substituted one,particularly the t -Bu-MeO-BIPHEP 4h could get 99%conversion with 97%ee (Table 1,entry 7).These results clearly indicated the strong influence of para -substituent with steric hindrance on the enantioselectivity of the reaction.With the optimal reaction conditions in hand,ligand 4h was used in the hydrogenation of a series of acetophenones derivatives (Table 2,entries 1–10).Excellent enantioselectivities ee were observed.The best result was hydrogenation of o -bromoacetophenone (Table 2,entry 7),in which the conversion to products was >99%with 99%ee.The o -chloroacetophenone (Table 2,entry 8)and o -trifluoromethylacetophenone (Table 2,entry 6)could get better ee at 97%.These results are comparable to the best results reported to date.In summary,we have investigated the influence of the para -substituent in the asymmetric hydrogenation of ketones,the results showed that new ruthenium catalysts [diphosphine RuCl 2diamine]containing the p -t -Bu-or p -i -Pr-substituted MeO-BIPHEP ligand were better than non-substituted ones in both activities and enantioselectivities.Further study will be focused on the synthesis and applications of other ortho -substituted MeO-BIPHEP ligands.M.L.Ma et al./Chinese Chemical Letters 21(2010)576–579578Scheme 2.Reagents and conditions:(a)DMF and [Ru(benzene)Cl 2]21008C,25min and (b)(S,S )-DPEN,258C,5h.The solvent DMF was removed by evaporated at 308C under reduced pressure.Table 1The effect of the para -alkoxy chain in the hydrogenation ofacetophenone..Entry Phosphine R 1R 2Con.(%)ee (%)a 14a CH 3H 9981b 24c CH 3H 998234d CH 3H >998444e CH 3H >998454f CH 3H >999264g CH 3H >998774h CH 3H >999784iCH 3H2977aReactions were run under 3Mp H 2and 208C in 50mL magnetically stirred Parr pressure vessels and in 12h.S/C/B 3000/1/100.Conversion and ees were determined by GC analysis (Chirasil DEX-CB column).bHenschke et al.reported 84%ee using RuCl 2[(S )-MeO-BIPHEP][(S,S )-DPEN]precatalyst and t -BuOK as base [7].M.L.Ma et al./Chinese Chemical Letters21(2010)576–579579 Table2The results of hydrogenation different acetophenones derivatives.Entry Phosphine R1R2Con.(%)ee(%) 1t-Bu-MeO-BIPHEP(4h)CH2CH3H>99822CH2(CH3)2H19783CH3p-CH3O89744CH3p-CF3>99845CH3o-CH3O53476CH3o-CF3>99987CH3o-Br>99998CH3o-Cl>99979CH3o-F986410CH3o-OH00 Reactions were run under3Mp H2,208C in50mL magnetically stirred Parr pressure vessels and in12h.S/C/B3000/1/100.Conversion and ees were determined by GC analysis(Chirasil DEX-CB column).AcknowledgmentsWe thank the NSFC(No.20272037),the Doctor’s Foundation of Education Ministry of China(No.20030610022) and Foundation of Xihua University(No.R0723315)and(No.07ZA109)for thefinancial support of this work. References[1](a)W.J.Tang,X.M.Zhang,Chem.Rev.103(2003)3029;(b)M.MacCarthy,P.J.Guiry,Tetrahedron57(2001)3809;(c)X.M.Zhang,Enantiomer4(1999)541.[2](a)R.Noyori,Angew.Chem.,Int.Ed.41(2002)2008;(b)R.Noyori,T.Ohkuma,Angew.Chem.,Int.Ed40(2001)40;(c)Z.G.Antonio,H.William,G.Michelle,et al.Platinum Met.Rev.49(2005)158.[3]P.Dotta,A.Magistrato,U.Rothlisberger,et anometallics21(2002)3033.[4]T.Ohkuma,M.Koizumi,K.Muniz,et al.J.Am.Soc.Chem.124(2002)6508.[5](a)J.Wu,H.Chen,W.Kwok,et .Chem.67(2002)7908;(b)J.Wu,J.X.Ji,R.Guo,et al.Eur.J.Chem.9(2003)2963.[6]B.H.Lipshutz,K.Noson,W.Chrisman,et al.J.Am.Soc.Chem.125(2003)8779.[7](a)J.P.Henschke,Z.G.Antonio,P.Moran,et al.Tetrahedron Lett.44(2003)4379;(b)J.P.Henschke,M.J.Burk,C.G.Malan,Adv.Synth.Catal.345(2003)300.[8](a)R.Schmid,E.A.Broger,M.Cereghetti,Pure Appl.Chem.68(1996)131;(b)E.A.Broger,J.Foricher,R.Schmid,US5,274,125,1993,Chem.Abstr.1993,118,P59878a;;(c)J.Foricher,B.Heiser,R.Schmid,US5,302,738,1994,Chem.Abstr.1993,118,P147774u;;(d)londe,5,536,858,1996,Chem.Abstr.1996,124,P8995c.[9]M.L.Ma,Z.H.Peng,H.Chen,J.Chin.Chem.24(2006)1391.[10]H.Doucet,T.Okhuma,R.Noyori,Angew.Chem.,Int.Ed.37(1998)1703.。
全球天然氢气勘探开发利用进展及中国的勘探前景
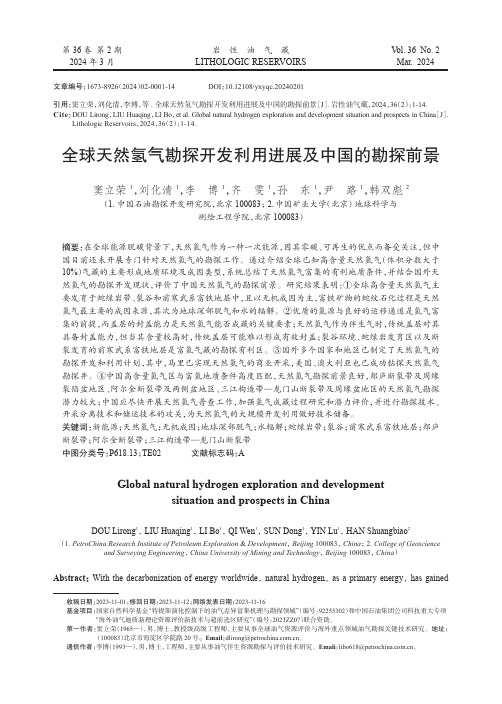
第36卷第2期2024年3月岩性油气藏LITHOLOGIC RESERVOIRSV ol.36No.2Mar.2024收稿日期:2023-11-01;修回日期:2023-11-12;网络发表日期:2023-11-16基金项目:国家自然科学基金“特提斯演化控制下的油气差异富集机理与勘探领域”(编号:92255302)和中国石油集团公司科技重大专项“海外油气地质新理论资源评价新技术与超前选区研究”(编号:2023ZZ07)联合资助。
第一作者:窦立荣(1965—),男,博士,教授级高级工程师,主要从事全球油气资源评价与海外重点领域油气勘探关键技术研究。
地址:(100083)北京市海淀区学院路20号。
Email :**********************.cn 。
通信作者:李博(1993—),男,博士,工程师,主要从事油气伴生资源勘探与评价技术研究。
Emali :**********************.cn 。
文章编号:1673-8926(2024)02-0001-14DOI :10.12108/yxyqc.20240201引用:窦立荣,刘化清,李博,等.全球天然氢气勘探开发利用进展及中国的勘探前景[J ].岩性油气藏,2024,36(2):1-14.Cite :DOU Lirong ,LIU Huaqing ,LI Bo ,et al.Global natural hydrogen exploration and development situation and prospects in China [J ].Lithologic Reservoirs ,2024,36(2):1-14.全球天然氢气勘探开发利用进展及中国的勘探前景窦立荣1,刘化清1,李博1,齐雯1,孙东1,尹路1,韩双彪2(1.中国石油勘探开发研究院,北京100083;2.中国矿业大学(北京)地球科学与测绘工程学院,北京100083)摘要:在全球能源脱碳背景下,天然氢气作为一种一次能源,因其零碳、可再生的优点而备受关注,但中国目前还未开展专门针对天然氢气的勘探工作。
酸化吹气-重铬酸钾法测定高氯地表水中化学需氧量
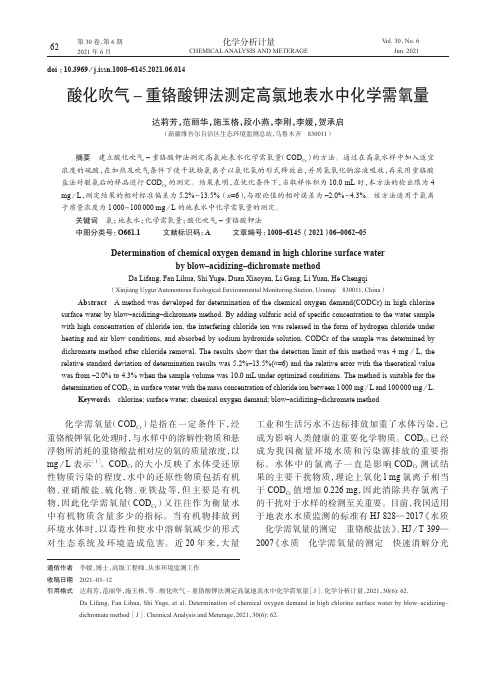
化学分析计量CHEMICAL ANALYSIS AND METERAGE第30卷,第6期2021年6月V ol. 30,No. 6Jun. 202162doi :10.3969/j.issn.1008–6145.2021.06.014酸化吹气–重铬酸钾法测定高氯地表水中化学需氧量达莉芳,范丽华,施玉格,段小燕,李刚,李媛,贺承启(新疆维吾尔自治区生态环境监测总站,乌鲁木齐 830011)摘要 建立酸化吹气–重铬酸钾法测定高氯地表水化学需氧量(COD Cr )的方法。
通过在高氯水样中加入适宜浓度的硫酸,在加热及吹气条件下使干扰物氯离子以氯化氢的形式释放出,并用氢氧化钠溶液吸收,再采用重铬酸盐法对驱氯后的样品进行COD Cr 的测定。
结果表明,在优化条件下,当取样体积为10.0 mL 时,本方法的检出限为4 mg /L ,测定结果的相对标准偏差为5.2%~13.5%(n =6),与理论值的相对误差为–2.0%~4.3%。
该方法适用于氯离子质量浓度为1 000~100 000 mg /L 的地表水中化学需氧量的测定。
关键词 氯;地表水;化学需氧量;酸化吹气–重铬酸钾法中图分类号:O661.1 文献标识码:A 文章编号:1008–6145(2021)06–0062–05Determination of chemical oxygen demand in high chlorine surface waterby blow–acidizing–dichromate methodDa Lifang, Fan Lihua, Shi Yuge, Duan Xiaoyan, Li Gang, Li Yuan, He Chengqi(Xinjiang Uygur Autonomous Ecological Environmental Monitoring Station, Urumqi 830011, China )Abstract A method was developed for determination of the chemical oxygen demand(CODCr) in high chlorine surface water by blow–acidizing–dichromate method. By adding sulfuric acid of speci fic concentration to the water sample with high concentration of chloride ion, the interfering chloride ion was released in the form of hydrogen chloride under heating and air blow conditions, and absorbed by sodium hydroxide solution. CODCr of the sample was determined by dichromate method after chloride removal. The results show that the detection limit of this method was 4 mg /L, the relative standard deviation of determination results was 5.2%–13.5%(n =6) and the relative error with the theoretical value was from –2.0% to 4.3% when the sample volume was 10.0 mL under optimized conditions. The method is suitable for the determination of COD Cr in surface water with the mass concentration of chloride ion between 1 000 mg /L and 100 000 mg /L.Keywords chlorine; surface water; chemical oxygen demand; blow–acidizing–dichromate method化学需氧量(COD Cr )是指在一定条件下,经重铬酸钾氧化处理时,与水样中的溶解性物质和悬浮物所消耗的重铬酸盐相对应的氧的质量浓度,以 mg /L 表示[1]。
HOMOGENEOUS ASYMMETRIC HYDROGENATION CATALYST

专利名称:HOMOGENEOUS ASYMMETRICHYDROGENATION CATALYST发明人:SHIMIZU, Hideo,IGARASHI,Daisuke,KURIYAMA, Wataru,YUSA, Yukinori 申请号:JP2006313510申请日:20060706公开号:WO07/007646P1公开日:20070118专利内容由知识产权出版社提供摘要:Disclosed is a homogeneous hydrogenation catalyst, in particular a homogeneous asymmetric hydrogenation catalyst containing an asymmetric copper complex having a chiral ligand. This catalyst is useful for hydrogenation reactions, particularly for asymmetric hydrogenation reactions. In addition, this catalyst is available comparatively easily and economical, while having good workability. Also disclosed is a method for producing a hydride of an unsaturated compound, particularly a method for producing an optically active compound having high optical purity with high yield by using the above-described catalyst.申请人:SHIMIZU, Hideo,IGARASHI, Daisuke,KURIYAMA, Wataru,YUSA, Yukinori地址:37-1, Kamata 5-chome, Ohta-ku, Tokyo 1448721 JP,c/o TAKASAGO INTERNATIONAL CORPORATION, Central Research Laboratory, 4-11, Nishiyawata 1-chome, Hiratsuka-shi, Kanagawa 2540073 JP,c/o TAKASAGO INTERNATIONAL CORPORATION, Central Research Laboratory, 4-11, Nishiyawata 1-chome, Hiratsuka-shi, Kanagawa 2540073 JP,c/o TAKASAGO INTERNATIONAL CORPORATION, Central Research Laboratory, 4-11, Nishiyawata 1-chome, Hiratsuka-shi, Kanagawa 2540073 JP,c/oTAKASAGO INTERNATIONAL CORPORATION, Central Research Laboratory, 4-11, Nishiyawata 1-chome, Hiratsuka-shi, Kanagawa 2540073 JP国籍:JP,JP,JP,JP,JP代理机构:IWATANI, Ryo更多信息请下载全文后查看。
《文献信息检索与利用》考试试题答案
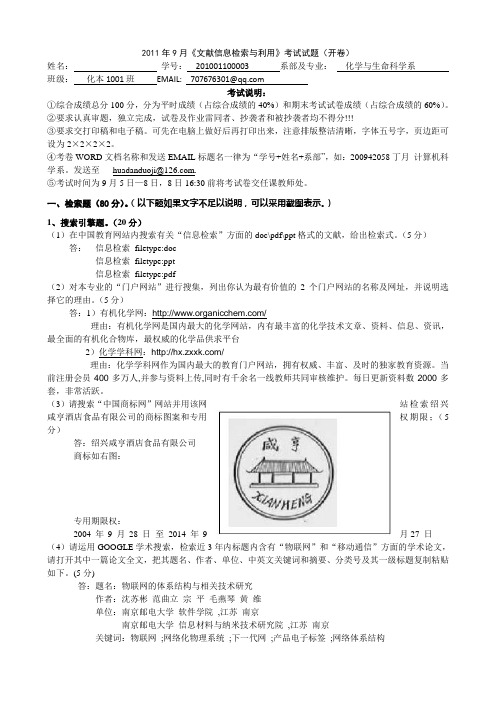
考试说明:①综合成绩总分100分,分为平时成绩(占综合成绩的40%)和期末考试试卷成绩(占综合成绩的60%)。
②要求认真审题,独立完成,试卷及作业雷同者、抄袭者和被抄袭者均不得分!!!③要求交打印稿和电子稿。
可先在电脑上做好后再打印出来,注意排版整洁清晰,字体五号字,页边距可设为2×2×2×2。
④考卷WORD文档名称和发送EMAIL标题名一律为“学号+姓名+系部”,如:200942058丁月_计算机科学系。
发送至huadanduoji@.⑤考试时间为9月5日—8日,8日16:30前将考试卷交任课教师处。
一、检索题(80分)。
(以下题如果文字不足以说明,可以采用截图表示。
)1、搜索引擎题。
(20分)(1)在中国教育网站内搜索有关“信息检索”方面的doc\pdf\ppt格式的文献,给出检索式。
(5分)答:信息检索filetype:doc信息检索filetype:ppt信息检索filetype:pdf(2)对本专业的“门户网站”进行搜集,列出你认为最有价值的2个门户网站的名称及网址,并说明选择它的理由。
(5分)答:1)有机化学网:/理由:有机化学网是国内最大的化学网站,内有最丰富的化学技术文章、资料、信息、资讯,最全面的有机化合物库,最权威的化学品供求平台2)化学学科网:/理由:化学学科网作为国内最大的教育门户网站,拥有权威、丰富、及时的独家教育资源。
当前注册会员400多万人,并参与资料上传,同时有千余名一线教师共同审核维护。
每日更新资料数2000多套,非常活跃。
(3)请搜索“中国商标网”网站并用该网站检索绍兴咸亨酒店食品有限公司的商标图案和专用权期限;(5分)答:绍兴咸亨酒店食品有限公司商标如右图:专用期限权:2004 年9 月28 日至2014 年9 月27 日(4)请运用GOOGLE学术搜索,检索近3年内标题内含有“物联网”和“移动通信”方面的学术论文,请打开其中一篇论文全文,把其题名、作者、单位、中英文关键词和摘要、分类号及其一级标题复制粘贴如下。
纳米压痕 纯铁 氢 硬度 位错

Recent developments in the study of hydrogen embrittlement:Hydrogeneffect on dislocation nucleationAfrooz Barnoush *,Horst VehoffSaarland University,Department of Materials Science,Bldg.D22,P.O.Box 151150,D-66041Saarbruecken,GermanyReceived 11March 2010;received in revised form 30May 2010;accepted 30May 2010Available online 2July 2010AbstractIn this paper,the intrinsic complexities of the experimental examination of hydrogen embrittlement are discussed.On the basis of these complexities,an experimental approach,in situ electrochemical nanoindentation,is proposed and performed on different materials.This technique is capable of registering the onset of plasticity in extremely small volumes,namely perfect crystals in hydrogen-free and charged conditions.It is shown that hydrogen reduces the required stress for the onset of plasticity,i.e.homogeneous dislocation nucle-ation by reduction in the shear modulus,dislocation line energy and stacking fault energy.The change in the shear modulus can be related to reduction in crystal cohesion whereas the reduction in dislocation line energy and stacking fault energy are explained by the defactant concept,i.e.reduction in the defect formation energy in the presence of hydrogen.Thus,neither hydrogen-enhanced dec-ohesion nor hydrogen-enhanced plasticity,but the reduction in the cohesion and defect formation energy are responsible for hydrogen embrittlement.Ó2010Acta Materialia Inc.Published by Elsevier Ltd.All rights reserved.Keywords:Hydrogen embrittlement;Nanoindentation;Yield phenomena;Electrochemistry;Dislocation nucleation1.IntroductionHydrogen embrittlement is a severe environmental type of failure that affects almost all metals and alloys.With advancing technology,the use of high-strength structural materials for lightweight construction and energy conser-vation becomes a necessity.In spite of the success of mate-rials scientists in developing alloys with outstanding combinations of high tensile strength and high fracture toughness,hydrogen embrittlement still has a widespread effect that severely degrades the fracture resistance of these alloys.On the other hand,with the depletion of fossil fuels,mankind is searching for other sources of energy.Hydro-gen is believed to be a possible future energy source and it is very possible that a “hydrogen economy ”will be real-ized within the next 50years.In such a scenario,large-scale production,storage,transportation and use of hydrogenwill become necessary.However,the problems in materials caused by hydrogen embrittlement,reported as early as 1875by Johnson [1],could limit the progress of such an economy.Since Johnson first reported on hydrogen embrittlement,various strong views on the mechanisms of hydrogen embrittlement have been vigorously discussed and thoroughly reviewed in the literature [2–4].Because of the technological importance of hydrogen embrittlement,many people have explored the nature,causes and control of hydrogen-related degradation of metals [5–7].This has resulted in an enormous number of sometimes controver-sial findings and/or interpretations.Moreover,frequently an ad hoc approach,focusing on urgent technical problems at hand,is adopted which raises the question of how this isolated case or interpretation really reflects on any basic,general concepts.It thus seems apparent that the findings gathered so far cannot be accounted for by a single domi-nant mechanism.As an example,Fig.1shows the results of conventional hydrogen embrittlement testing of 34different grades of1359-6454/$36.00Ó2010Acta Materialia Inc.Published by Elsevier Ltd.All rights reserved.doi:10.1016/j.actamat.2010.05.057*Corresponding author.E-mail address:a.barnoush@matsci.uni-sb.de (A.Barnoush)./locate/actamatActa Materialia 58(2010)5274–5285steel within465test runs[8].The embrittlement index shown in Fig.1is the reduction in the fracture area in hydrogen-charged vs.hydrogen-free conditions during uni-axial tensile tests.The scatter observed within the results shown in Fig.1demonstrates the need for a new experi-mental approach to the investigation of hydrogen embrit-tlement.It is necessary tofind the source of this scatter in conventional hydrogen embrittlement testing methods. In this paper we will briefly review the different experimen-tal approaches used to study hydrogen embrittlement with a special focus on in situ local examination methods of hydrogen–dislocation interactions due to the conclusive role of dislocations in fracture and hydrogen embrittlement aspect is the wide range of intrinsic/extrinsic variables con-cerning the material itself.The experimental approach for examination of hydrogen embrittlement is based on the elimination of diverse factors within these three aspects. For example,let us consider uniaxial straining of a single crystal sample combined with controlled hydrogen charg-ing on the surface.The uniaxial stressfield simplifies the stress condition,i.e.the mechanical aspect of hydrogen embrittlement,in the e of a single crystal and con-trolled hydrogen charging condition reduces the complexi-ties due to the material and environmental aspects of hydrogen embrittlement in the test.Unfortunately,due to the large difference in the scale of these aspects controlling the process of hydrogen embrittlement,this experimental methodology is not very successful.The uniaxial stressfield of the above-mentioned example is not uniform,unlike the scale of hydrogen interacting with defects like dislocations and vacancies.This problem becomes more complicated as the microstructure becomes more complex with the intro-duction of grain boundaries,pores,second phases,etc. On the other hand,surface hydrogen charging of macro-scopic samples results in a concentration gradient,which again can override the microscopic process of hydrogen embrittlement.Additionally,microstructural features like dislocations,grain boundaries and interfaces can interact with hydrogen diffusion by providing faster diffusion routes or acting as traps for hydrogen.Superimpose upon this theFig. 1.The hydrogen embrittlement index measured by conventionalmechanical testing for34different grades of steel within465test runsFig.2.Global description of hydrogen embrittlement interaction aspects.Materialia58(2010)5274–52855275grain boundary fracture,enhanced shear or enhanced localized microvoid formation,or all of the above.One possible solution to these problems,arising from the experimental methodology used for studying hydrogen embrittlement,is the local examination of the hydrogen effect.This entails reducing the scale of the testing method down to the microstructural level such that all three con-trolling aspects of hydrogen embrittlement(Fig.2)can be defined within the test.Few attempts of this kind of approach have been done previously.Vehoffand Neumann [9]developed a method for studying crack propagation in a controlled environment under controlled plastic strain con-ditions.This method was used to study the hydrogen effect on crack growth mechanisms in Fe–2.6%Si[10]and Ni [11].In their experiments they controlled:(i)environmental aspects by using an ultrahigh-vacuum chamber and con-trolling the partial pressure of hydrogen;(2)mechanical aspects by controlling plastic strain and confining the stress to a smallfield ahead of the crack;and(iii)material aspects by selection of specifically oriented single crystals and focusing on the fracture process zone(Fig.3).Through these perfectly defined experimental conditions they were able to propose a model based on hydrogen-enhanced dec-ohesion(HEDE)and hydrogen trapping in front of a crack tip in the fracture process zone[10,11].Another intelligent experimental approach for small-scale observation of hydrogen effects on mechanical prop-erties was use of in situ straining tests in an environmental transmission electron microscope(TEM)developed by Birnbaum and Sofronis[12].With this technique it was possible for thefirst time to directly observe the effect of hydrogen on dislocations.Unfortunately,there are some uncertainties within the experimental conditions of this technique which must be mentioned here:(i)The electron beam dissociates hydrogen molecules to produce atomic hydrogen.Bond et al.[13]determined that the fugacity of the dissociated hydrogen was between30and750MPa. (ii)Since mode III(shear off)is usually easier in thinfilms, crack growth experiments under a defined mode I loading are very difficult to realize and therefore interpretation of the crack growth results become very difficult.(iii)Field of view is limited to the transparent region of the sample.By the introduction of micro-and nanoscale mechanical testing methods,like instrumented nanoindentation(NI) and nanoindenting atomic force microscopy(NI-AFM),a new era in mechanical testing of extremely small volumes started[14].Gerberich et al.[15]was thefirst one who men-tioned the potential application of micromechanical tests for the examination of hydrogen embrittlement.Since then, several researchers have used the NI technique to probe the effects of hydrogen on mechanical properties[16–20].The main advantage of the NI technique is its capability to resolve the dislocation nucleation in samples with low dis-location density[21,22].Additionally,analytical solutions for stress underneath the tip prior to dislocation nucleation within the elastic deformation are available,so the mechan-ical aspects of the experiments can be defined.The volume probed during the test is extremely small and can be char-acterized by means of different techniques,like electron backscatter diffraction(EBSD)and electron channeling contrast[23],and therefore,material aspects can be resolved.The referenced studies[16–20]used ex situ hydro-gen charging techniques which resulted in uncertaintiesFig.3.Hydrogen effect on the crack tip opening angle in:(a)vacuum and(b)hydrogen atmosphere.Sharpness of the crack tip in stressed Fe–3wt.%single crystal increases progressively with increasing hydrogen pressure[10].Table1Summary of the high-resolution testing methods used for local examination of hydrogen embrittlement. Experimental approach Different aspects of the hydrogen embrittlementMechanical Environmental MaterialIn situ crackpropagation test Defined and analyticallycan be treatedDefined Defined in the case of macroscopic single crystals withspecific orientation,very hard to apply on complexmicrostructuresIn situenvironmentalTEM tests Undefined Undefined due to dissociation of Hmolecules by electron beam(low pressure,very high fugacity)Defined with very high-resolution but only within thefield of viewNanoindentation on ex situ chargedsamples Before pop-in is definedand can analytically betreatedUndefined,due to out gassing andconcentration gradientPerfect defect-free crystalIn situ ECnanoindentation Before pop-in is definedand can analytically betreatedDefined Perfect defect-free crystalMaterialia58(2010)5274–5285within the hydrogen concentration especially near the sur-face of the sample where the mechanical testing was carried out.We solved this problem by performing in situ ECNI tests while the surface hydrogen concentration was kept constant by setting the surface electrochemical potential inside the proper electrolyte[24–26].Table1summarizes the local high-resolution techniques for examination of hydrogen embrittlement.A comparison of these methods shows the advantage of ECNI in provid-ing the required conditions to perform a defined experi-ment in accordance with different aspects of hydrogen embrittlement.In this paper,we report the results of ECNI tests on different metals with different hydrogen embrittle-ment susceptibilities.It will be shown that ECNI is capable of quantitatively resolving the sensitivity to hydrogen embrittlement.3.Experimental3.1.MaterialsThe materials used in this study and the electropolishing parameters and electrochemical hydrogen charging condi-tions for each are summarized in Tables2and3.The sin-gle-crystal specimens were cut by spark erosion from large single crystals with a misalignment of less than2°controlled by EBSD.The polycrystalline specimens were cut from samples with large grains a few millimeters in diameter which act like a single crystal during NI when all tests are performed in the same rge grains or single crystals eliminate the scatter in the data due to orien-tation differences.The samples were mechanically polished down to0.25l m.Afterwards,samples were annealed at 80%of the melting temperature in a vacuum greater than 10À6mbar for24h and cooled in the furnace in order to minimize the lattice defect density.Immediately before indentation the samples were electropolished according to the parameters given in Table2to have a well-defined reproducible surface condition.Special attention was paid to surface preparation since,as mentioned before,a defect-free and low-roughness surface is necessary in order to observe the dislocation nucleation during NI.When examined with an AFM,the sample surface had an root-mean-square roughness of less than1nm,and asperities or steps appeared infrequently on the surface.3.2.InstrumentationThe experiments were performed with a Hysitron Tribo-ScopeÒin conjunction with a Digital Instruments Nano-scope IIÒand a Hysitron TriboIndenterÒ.The indenter, designed especially for tests in liquid,had a Berkovich or conical diamond tip(see Table3).For the NI-AFM system, a three-electrode electrochemical setup with a platinum counter electrode and an Ag/AgCl reference electrode was developed,as shown schematically in Fig.4.Consequently, all the electrochemical potentials in this work are reported against an Ag/AgCl reference electrode.A Bank Elektronik TG97potentiostat was used to control the electrochemical potentials,and the electrochemical data were recorded on a PC using an AD–DA interface.The cell was made from Tef-lon e,and made tofit into the nanoindenter sample holder. Nanoindentation tests were made inside this electrochemi-cal cell while the sample was covered with approximately 2mm of electrolyte.The whole system was put into a cham-ber containing a protective atmosphere of nitrogen and helium in order to eliminate the oxygen effect in the electro-chemical reaction.The solution was injected from outside the chamber through a polyethylene tube connected to a MicroFil e pipette.There are additional concerns that arise when indenting in a liquid environment due to the capillary and buoyancyTable2Summary of the samples studied and their electropolishing conditions.Material Purity Microstructure Electropolishing conditionSolution Potential(V)Temperature(°C)Time(s) Copper Zone refined(111)H3PO4/ethanol202030 Aluminum99.991mm grains HClO4/ethanol40030Fe–3wt.%Si See Table41mm grains H2SO4/CH3OH121060FeAl(40at.%Al)Zone refined(100)H2SO4/CH3OH351090 Nickel Zone refined(111)H2SO4/CH3OH301560 Table3Summary of the electrochemical hydrogen charging and discharging(passivation)conditions.Material Test solution pH H-charging potential Passivation potential Indenter tip Copper Borate buffer9À1000mV250mV Berkovich Aluminum Borate buffer9À1250mV OCP Berkovich Aluminum0.05M Na2SO46OCP250mV Berkovich Fe–3wt.%Si Borate buffer9À1000mV n.a.Berkovich FeAl0.05M Na2SO46À1100mV100mV Conical Nickel0.05M Na2SO46À1000mV500mV BerkovichA.Barnoush,H.Vehoff/Acta Materialia58(2010)5274–52855277forces acting on the tip.To overcome this,the surface is first engaged with a higher set point of contact force.The tip is then moved a few nanometres away from the surface and the forces on the tip balance while it rests above the surface.After this procedure the surface can be easily engaged as usual.Another important concern during the in situ ECNI test is cleanliness.Therefore,all electrolytes were prepared from analytical grade compounds and dou-ble-distilled water.Prior to the tests,the electrochemical cell was cleaned in piranha acid to remove most organic matter and to make it hydrophilic and water compatible.The tip and its shaft were also cleaned carefully before each test in a mixture of ethanol and isopropanol and then in double-distilled water.A clean tip shaft reduces the capil-lary forces acting on it and reduces the probability of dis-engagement of the tip during surface imaging inside the solution.A low impurity content in the electrolyte inhibitsthe deposition of these impurities during electrochemical polarization of the surface.The electrolyte pH and compo-sition should also be selected very carefully to inhibit any possible corrosion and surface roughening.Hence,these parameters are selected for each sample individually.4.Results 4.1.CopperCopper is known for its very low hydrogen solubility and diffusivity.DeWulf and Bard [27]showed that without hydrogen recombination poisons (e.g.As 2O 3)no detectable hydrogen concentration develops in copper.This makes the copper a proper calibration material for in situ ECNI tests,where no effect of cathodic charging should be observed.Typical load–displacement (L –D )curves under cathodic and anodic potentials are given in Fig.5.4.2.AluminumAluminum is a reactive metal protected by a thin stable oxide layer with semi-conductive properties.At potentials cathodic to the open circuit potential (OCP)of Al,cathodic hydrogen evolution due to the reduction of hydrogen ions and water occurs simultaneously with anodic oxidation and dissolution processes [28].This makes the electrochem-ical hydrogen charging of Al without damaging the surface difficult.Therefore,an alternative method controlling the pH of the solution was developed and used for hydrogen charging of Al [29].It is well known that oxide-covered metals immersed in aqueous solutions terminate in an out-ermost layer of hydroxyl groups due to their interaction with water molecules [30].In aqueous solutions,the surface hydroxyl groups will remain undissociated if the pH of theTable 4Analysis of the Fe–3wt.%Si alloy used in this study in.C Si Mn P S Cr Ni Mo Cu Al Ti Nb V B Zr Ce 0.0032.3830.2020.0130.0120.0330.0480.0150.0200.3650.0050.0200.0020.00080.0050.009Fig.4.Schematic drawing of the experimental setup.10020030040050001020304050607080Depth (nm)01002003004005006000102030405060708090Depth (nm)Fig.5.Typical L –D curves of copper under:cathodic and (b)anodic polarization.Materialia 58(2010)5274–5285aqueous solution is the same as the isoelectric point (IEP)of the oxide.If the pH is less than the IEP,the surface will acquire a positive charge.MOH ðsurf ÞþH þðaq Þ!MOH þ2ðsurf Þð1ÞIf the pH is greater than the IEP,the surface will acquire a negative charge MOH ðsurf ÞþOH Àðaq Þ!MO Àðsurf ÞþH 2Oð2ÞThe air-formed oxide film on Al has the IEP value of9.5[31].According to the Eqs.(1)and (2),the pH can greatly affect the hydrogen absorption during immersion of the sample in electrolyte under OCP.Hence,the tests on Al were performed in two different solutions (Table 3).Typical L –D curves of the aluminum sample at different pH levels and electrochemical polarizations are given in Fig.6.4.3.Fe–3wt.%SiThe Fe–3wt.%Si alloy is a single-phase body-centered cubic crystal.The electrochemical behavior of this metal is quite similar to that of pure iron and has a very low aqueous corrosion resistance in solutions with low pH.Therefore,in situ ECNI tests were performed in a pH 9borate buffer solution.This electrolyte is preferred because of the possibility of both controlled cathodic removal of prior oxide films and highly efficient anodic passivation.However,during the in situ ECNI experiments,due to the limited volume of the electrochemical cell and the use of Ag/AgCl reference electrodes,contamination of electro-lyte with Cl Àions resulted in the breakdown of the passive layer.All attempts for producing a stable passive layer failed and resulted in a corroded surface that was not suit-able for NI experiments.Therefore,in the case of the Fe–3wt.%Si sample,the NI results under cathodic potential are compared with the results in air.Fig.7a shows typical load displacement curves during NI of the Fe–3wt.%Si sample in air.The same sample was indented in the same grain under a cathodic potential of À1000mV.This resulted in the load displacement curves shown in Fig.7b.4.4.FeAl intermetallicThe commercial importance of iron aluminides for high temperature structural applications has been well estab-lished [32].Iron aluminides contain two of the most widely available metals,namely Fe and Al.They offer low mate-rial costs,conservation of strategic materials and lower density than stainless steels,with excellent oxidation and sulfidation resistance.In spite of all these inherent advanta-ges,the binary iron aluminides suffer from hydrogen embrittlement.A conical indenter with a cone angle of 90°was used to perform the indentations on FeAl intermetallic alloy with 40at.%Al.The resulting L –D curves in different conditions are given in Fig.8.4.5.NickelThe freshly electropolished nickel has a thin (0.6–0.8nm)film of NiO which can be reduced by cathodic polarization in Na 2SO 4solution with a pH lower than 8[33].Anodic polarization of nickel in the same solution produces a new passive layer,which is a 0.9–1.2nm film of NiO.This film cannot be reduced in a neutral solution.This fact was used during in situ ECNI experiments to exclude surface effects from the effects of hydrogen [34].D curves of aluminum in:(a)pH6solution (OCP light-colored/anodic dark-colored)and (b)pH9solution (cathodic light-colored/OCP 05001000150020002500255075100Depth (nm)o a d (µN )H-chargedH-freeHertzian fitL –D curves of Fe–3wt.%Si in:(a)air and (b)hydrogen-charged condition.Typical load displacement curves obtained in different con-dition are shown in Fig.9.5.DiscussionThe L –D curves of Figs.5–9all show three stages:elastic loading,an excursion in depth (pop-in)at the onset of plas-ticity and continued elastoplastic loading.The initial elastic portion of the loading curves can be fitted with the Hertz-ian elastic responseP ¼43E r ffiffiffiffiffiffiffiffiRh 3p ð3Þwhere P is the applied load,h is the indentation depth,R is the radius of the tip curvature and E r is the reduced mod-ulus,given by 1E r ¼1Àm 21E 1þ1Àm 22E 2ð4ÞHere E is the elastic modulus of the material,m is the Poisson’s ratio,and the subscripts 1and 2refer to the tip and the sample respectively.The initial elastic loading begins as soon as the tip contacts the material surface and continues until dislocation nucleation or motion occurs.Typical dislocation densities in an annealed metal are in the range 106–108cm À2,with dislocations spaced between 1and 10l m apart.A typical indentation test in the elastic regime probes a lateral region of at most a couple of hun-dred nanometers to the point where the pop-in is noted.This suggests the volume of material sampled by the inden-tation test at this depth is smaller than the average disloca-tion spacing,so that an indentation placed randomly on the surface would have a significant probability of sampling a region that contains no pre-existing dislocations.Experi-mental studies [35,36]have been conducted and all lend credibility to the assumption that,for a well-annealed and electropolished sample,the indenter tip can initially contact a volume of material small enough to be dislocation free.The absence of dislocations means the material continues to load elastically until the shear stress under the tip reaches a value near the theoretical shear strength of the material,well above that necessary to activate an existing dislocation source.At this point dislocations are homogeneously nucle-ated,followed by subsequent glide and multiplication events.Homogeneous dislocation nucleation (HDN)should occur when the stress beneath the indenter tip approaches the theoretical shear strength of the material.The term HDN is used here to indicate that dislocations are nucleated from otherwise dislocation-free material.For an indentation test,the applied shear stress that nucle-ates a dislocation can be assumed to be the maximum shear stress beneath the indenter during purely elastic loading.According to continuum mechanics,the maximum shear stress is acting on a point 0.48times the contact radius,a ,below the sample puter simulations have also shown that dislocations nucleate at this point [37].The posi-tion of this maximum shear stress z s (max)and its value s max is givenbyFig.8.Typical L –D curves of FeAl in:(a)hydrogen-free and (b)hydrogen-charged conditions.0501001502002503000102030405060Depth (nm)Depth (nm)a10020030040050001020304050607080ba d (µN )a d (µN )Pop-inFig.9.L –D curves for nickel in:(a)hydrogen-charged and (b)hydrogen-free conditions.z sðmaxÞ¼0:48Áa¼0:483PR4E r13ð5Þs max¼0:316E2rp3RP13ð6ÞTable5summarizes the effect of hydrogen charging on the mean value of pop-in load s max and its position below the surface of all studied samples.For comparison,the the-oretical strength(s th%l/20)according to Frenkel’s model is also given in the Table5.5.1.Hydrogen effect on HDNThe above results clearly indicate that the pop-in load in the hydrogen-charged condition is lower than in the hydrogen-free condition.This change cannot be attributed to the surface topography since it has been imaged and revealed no surface roughening[24,25].Fig.10,as an example,shows the surface topography of the FeAl sam-ple inside the electrolyte.Therefore,the reduction in the pop-in load clearly shows the hydrogen effect on HDN. This is in good agreement with molecular dynamic simu-lations performed by Wen et al.[37].Their results,like other experimental NI results on hydrogen-charged mate-rials[18–20],used hydrogen-enhanced plasticity(HELP) to explain the reduction in the pop-in load.However, the analytical description of the HELP mechanism is based on reduction of elastic interactions by solute hydro-gen between dislocations and elastic centers which act as barriers,such as nearby dislocations and solute pinning points[12].As mentioned before,during NI,the probed volume of the material is so small that it can be assumed to be a perfect crystal without any defects.Therefore,elas-tic shielding of the dislocations by trapped hydrogen atoms is not capable of explaining the observed enhanced plasticity.In adsorption-induced dislocation emission (AIDE)[2,38]the formation energy of dislocations at the crack tip is reduced by hydrogen being absorbed on the crack surfaces.As opposed to HELP,it is the external hydrogen on the crack surface and not the internal hydro-gen in the bulk that enhances the dislocation nucleation in AIDE.Therefore,neither HELP(in the original formula-tion[12])nor AIDE is capable of explaining the hydro-gen-induced reduction in the pop-in load during NI.According to the classic dislocation theory,the free energy required for HDN during NI is determined by the line energy of the newly formed loop c dis and the work for extending the dislocation loop s b per loop area.Here s is the maximum shear stress under the indenter induced by the external load and b is the Burgers vector of the dis-location.If the dislocation forms as a partial,the stacking fault energy(SFE)c also contributes to the required energy for HDN.Then the formation energy of a circular loop of radius r isD G¼2p r cdisþp r2cÀp r2b sð7ÞThe elastic self-energy for a full circular dislocation loop of radius r in an infinite elastic solid is given byc dis¼2Àt1Àtl b2r4ln4rqÀ2ð8ÞFig.11shows the plots of D G as a function of loop radius calculated for the observed mean values of s max in the hydrogen-charged and hydrogen-free conditions.The material constants used are given in Tables5and6.The free-energy curves for HDN pass through a maximumTable5Summary of the mean pop-in load for the H-charged and H-free conditions and comparison between theoretical strength,l/20and measured shear stress at the onset of the pop-in.3topography of FeAl sample under:(a)cathodic and(b)anodic potential where no surface rougheningA.Barnoush,H.Vehoff/Acta Materialia58(2010)5274–52855281。
高效液相色谱法测定人血浆中左乙拉西坦的药物浓度
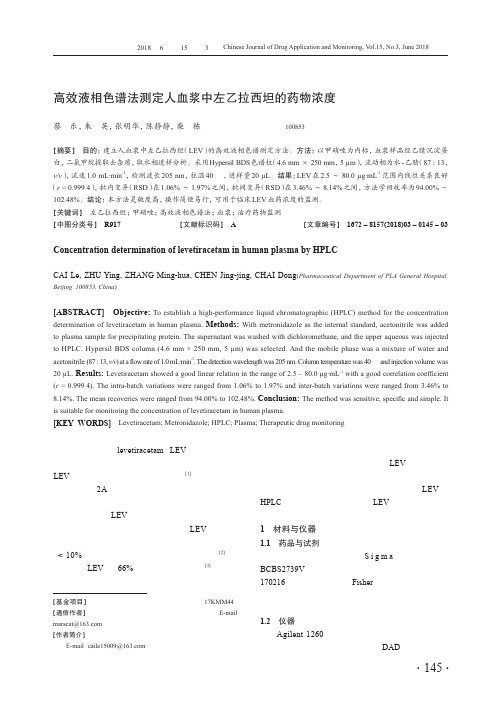
高效液相色谱法测定人血浆中左乙拉西坦的药物浓度蔡 乐,朱 英,张明华,陈静静,柴 栋(解放军总医院药学部,北京 100853)[摘要] 目的:建立人血浆中左乙拉西坦(LEV)的高效液相色谱测定方法。
方法:以甲硝唑为内标,血浆样品经乙腈沉淀蛋白,二氯甲烷提取去杂质,取水相进样分析。
采用Hypersil BDS色谱柱(4.6 mm × 250 mm,5 µm),流动相为水-乙腈(87 : 13,v/v),流速1.0 mL·min-1,检测波长205 nm,柱温40 ℃,进样量20 µL。
结果:LEV在2.5 ~ 80.0 µg·mL-1范围内线性关系良好(r = 0.999 4),批内变异(RSD)在1.06% ~ 1.97%之间,批间变异(RSD)在3.46% ~ 8.14%之间,方法学回收率为94.00% ~102.48%。
结论:本方法灵敏度高,操作简便易行,可用于临床LEV血药浓度的监测。
[关键词] 左乙拉西坦;甲硝唑;高效液相色谱法;血浆;治疗药物监测[中图分类号] R917 [文献标识码] A [文章编号] 1672 – 8157(2018)03 – 0145 – 03 Concentration determination of levetiracetam in human plasma by HPLCCAI Le, ZHU Ying, ZHANG Ming-hua, CHEN Jing-jing, CHAI Dong(Pharmaceutical Department of PLA General Hospital, Beijing 100853, China)[ABSTRACT] Objective: To establish a high-performance liquid chromatographic (HPLC) method for the concentration determination of levetiracetam in human plasma. Methods: With metronidazole as the internal standard, acetonitrile was added to plasma sample for precipitating protein. The supernatant was washed with dichloromethane, and the upper aqueous was injected to HPLC. Hypersil BDS column (4.6 mm × 250 mm, 5 µm) was selected. And the mobile phase was a mixture of water and acetonitrile (87 : 13, v/v) at a flow rate of 1.0 mL·min-1. The detection wavelength was 205 nm. Column temperature was 40 ℃ and injection volume was 20 µL. Results: Levetiracetam showed a good linear relation in the range of 2.5 – 80.0 µg·mL-1 with a good correlation coefficient (r = 0.999 4). The intra-batch variations were ranged from 1.06% to 1.97% and inter-batch variations were ranged from 3.46% to 8.14%. The mean recoveries were ranged from 94.00% to 102.48%. Conclusion: The method was sensitive, specific and simple. It is suitable for monitoring the concentration of levetiracetam in human plasma.[KEY WORDS] Levetiracetam; Metronidazole; HPLC; Plasma; Therapeutic drug monitoring左乙拉西坦(levetiracetam,LEV)是一种吡咯烷酮衍生物,其化学结构与其他抗癫痫药物无相关性。
二叠纪 三叠纪

二叠纪-三叠纪二叠纪-三叠纪灭绝事件(Permian–Triassic extinction event)是一个大规模物种灭绝事件,发生于古生代二叠纪与中生代三叠纪之间,距今大约2亿5140万年前。
[1][2]若以消失的物种来计算,当时地球上70%的陆生脊椎动物,以及高达96%的海中生物消失;[3]这次灭绝事件也造成昆虫的唯一一次大量灭绝,计有57%的科与83%的属消失。
[4][5]在灭绝事件之后,陆地与海洋的生态圈花了数百万年才完全恢复,比其他大型灭绝事件的恢复时间更长久。
[3]此次灭绝事件是地质年代的五次大型灭绝事件中,规模最庞大的一次,因此又非正式称为大灭绝(Great Dying),或是大规模灭绝之母(Mother of all mass extinctions)。
[6]二叠纪-三叠纪灭绝事件的过程与成因仍在争议中。
[7]根据不同的研究,这次灭绝事件可分为一[1]到三[8]个阶段。
第一个小型高峰可能因为环境的逐渐改变,原因可能是海平面改变、海洋缺氧、盘古大陆形成引起的干旱气候;而后来的高峰则是迅速、剧烈的,原因可能是撞击事件、火山爆发、或是海平面骤变,引起甲烷水合物的大量释放。
[9]年代测定在公元2000年之前,二叠纪与三叠纪交界的地层很少被发现,因此科学家们很难准确地估算灭绝事件的年代与经历时间,以及影响的地理范围。
[10]在1998年,科学家研究中国浙江省长兴县煤山附近的二叠纪/三叠纪岩层,他们采用铀-铅测年方法,研究锆石中的铀/铅比例,[2]估计二叠纪-三叠纪灭绝事件的发生年代为2亿5140万年前(误差值为30万年),并发现此后有随者时间持续增高的灭绝比例。
[1]在灭绝事件发生时,全球各地的碳13/碳12比例极速下降约9‰。
[11][12][13][14][15]因为二叠纪/三叠纪的界线难以用放射性定年法测定,科学家们多用急遽下降的碳13/碳12比例,测定岩层中的二叠纪/三叠纪交界。
标准红外光谱图谱

Go to: home • ir • proton nmr • carbon nmr• mass specTable of Contents - IRI. HydrocarbonsII. Halogenated HydrocarbonsIII. Nitrogen Containing CompoundsIV. Silicon Containing Compounds (Except Si-O)V. Phosphorus Containing Compounds (Except P-O And P(=O)-O) VI. Sulfur Containing CompoundsVII. Oxygen Containing Compounds (Except -C(=O)-)VIII. Compounds Containing Carbon To Oxygen Double BondsI. HydrocarbonsA. Saturated Hydrocarbons1. Normal Alkanes2. Branched Alkanes3. Cyclic AlkanesB. Unsaturated Hydrocarbons1. Acyclic Alkenes2. Cyclic Alkenes3. AlkynesC. Aromatic Hydrocarbons1. Monocyclic (Benzenes)2. PolycyclicII. Halogenated HydrocarbonsA. Fluorinated Hydrocarbons1. Aliphatic2. AromaticB. Chlorinated Hydrocarbons1. Aliphatic2. Olefinic3. AromaticC. Brominated Hydrocarbons1. Aliphatic2. Olefinic3. AromaticD. Iodinated Hydrocarbons1. Aliphatic and Olefinic2. AromaticIII. Nitrogen Containing CompoundsA. Amines1. Primarya. Aliphatic and Olefinicb. Aromatic2. Secondarya. Aliphatic and Olefinicb. Aromatic3. Tertiarya. Aliphatic and Olefinicb. AromaticB. PyridinesC. QuinolinesD. Miscellaneous Nitrogen HeteroaromaticsE. HydrazinesF. Amine SaltsG. Oximes (-CH=N-OH)H. Hydrazones (-CH=N-NH2)I. Azines (-CH=N-N=CH-)J. Amidines (-N=CH-N)K. Hydroxamic AcidsL. Azo Compounds (-N=N-)M. Triazenes (-N=N-NH-)N. Isocyanates (-N=C=O)O. Carbodiimides (-N=C=N-)P. Isothiocyanates (-N=C=S)Q. Nitriles (-C≡N)1. Aliphatic2. Olefinic3. AromaticR. Cyanamides (=N-C≡N)S. Thiocyanates (-S-C≡N)T. Nitroso Compounds (-N=O)U. N-Nitroso Compounds (=N-N=O)V. Nitrites (-O-N=O)W. Nitro Compounds (-NO2)1. Aliphatic2. AromaticX. N-Nitro-Compounds (=N-NO2)IV. Silicon Containing Compounds (Except Si-O)V. Phosphorus Containing Compounds (Except P-O and P(=O)-O) VI. Sulfur Containing CompoundsA. Sulfides (R-S-R)1. Aliphatic2. Heterocyclic3. AromaticB. Disulfides (R-S-S-R)C. Thiols1. Aliphatic2. AromaticD. Sulfoxides (R-S(=O)-R)E. Sulfones (R-SO2-R)F. Sulfonyl Halides (R-SO2-X)G. Sulfonic Acids (R-SO2-OH)1. Sulfonic Acid Salts (R-SO2-O-M)2. Sulfonic Acid Esters (R-SO2-O-R)3. Sulfuric Acid Esters (R-O-S(=O)-O-R)H. Thioamides (R-C(=S)-NH2)I. Thioureas (R-NH-C(=S)-NH2)J. Sulfonamides (R-SO2-NH2)K. Sulfamides (R-NH-SO2-NH-R)VII. Oxygen Containing Compounds (Except -C(=O)-)A. Ethers1. Aliphatic Ethers (R-O-R)2. Acetals (R-CH-(-O-R)2)3. Alicyclic Ethers4. Aromatic Ethers5. Furans6. Silicon Ethers (R3-Si-O-R)7. Phosphorus Ethers ((R-O)3-P)8. Peroxides (R-O-O-R)B. Alcohols (R-OH)1. Primarya. Aliphatic and Alicyclicb. Olefinicc. Aromaticd. Heterocyclic2. Secondarya. Aliphatic and Alicyclicb. Olefinicc. Aromatic3. Tertiarya. Aliphaticb. Olefinicc. Aromatic4. Diols5. Carbohydrates6. PhenolsVIII. Compounds Containing Carbon To Oxygen Double BondsA. Ketones (R-C(=O)-R)1. Aliphatic and Alicyclic2. Olefinic3. Aromatic4. α-Diketones and β-DiketonesB. Aldehydes (R-C(=O)-H)C. Acid Halides (R-C(=O)-X)D. Anhydrides (R-C(=O)-O-C(=O)-R)E. Amides1. Primary (R-C(=O)-NH2)2. Secondary (R-C(=O)-NH-R)3. Tertiary (R-C(=O)-N-R2)F. Imides (R-C(=O)-NH-C(=O)-R)G. Hydrazides (R-C(=O)-NH-NH2)H. Ureas (R-NH-C(=O)-NH2)I. Hydantoins, Uracils, BarbituratesJ. Carboxylic Acids (R-C(=O)-OH)1. Aliphatic and Alicyclic2. Olefinic3. Aromatic4. Amino Acids5. Salts of Carboxylic AcidsK. Esters1. Aliphatic Esters of Aliphatic Acids2. Olefinic Esters of Aliphatic Acids3. Aliphatic Esters of Olefinic Acids4. Aromatic Esters of Aliphatic Acids5. Esters of Aromatic Acids6. Cyclic Esters (Lactones)7. Chloroformates8. Esters of Thio-Acids9. Carbamates10. Esters of Phosphorus AcidsPublished by Bio-Rad Laboratories, Inc., Informatics Division. © 1978-2004 Bio-Rad Laboratories, Inc. All Rights Reserved.Go to: home • ir • proton nmr • carbon nmr• mass specTable of Contents - Proton NMRI. HydrocarbonsII. Halogenated HydrocarbonsIII. Nitrogen Containing CompoundsIV. Silicon Containing Compounds (Except Si-O)V. Phosphorus Containing Compounds (Except P-O and P(=O)-O) VI. Sulfur Containing CompoundsVII. Oxygen Containing Compounds (Except -C(=O)-)VIII. Compounds Containing Carbon To Oxygen Double BondsI. HydrocarbonsA. Saturated Hydrocarbons1. Normal Alkanes2. Branched Alkanes3. Cyclic AlkanesB. Unsaturated Hydrocarbons1. Acyclic Alkenes2. Cyclic Alkenes3. AlkynesC. Aromatic Hydrocarbons1. Monocyclic (Benzenes)2. PolycyclicII. Halogenated HydrocarbonsA. Fluorinated Hydrocarbons1. Aliphatic2. AromaticB. Chlorinated Hydrocarbons1. Aliphatic2. AromaticC. Brominated Hydrocarbons1. Aliphatic2. AromaticD. Iodinated Hydrocarbons1. Aliphatic2. AromaticIII. Nitrogen Containing CompoundsA. Amines1. Primarya. Aliphaticb. Aromatic2. Secondarya. Aliphaticb. Aromatic3. Tertiarya. Aliphaticb. AromaticB. PyridinesC. Quaternary Ammonium SaltsD. HydrazinesE. Amine SaltsF. Ylidene Compounds (-CH=N-)G. Oximes (-CH=N-OH)H. Hydrazones (-CH=N-NH2)I. Azines (-CH=N-N=CH-)J. Amidines (-N=CH-N)K. Hydroxamic AcidsL. Azo Compounds (-N=N-)M. Isocyanates (-N=C=O)N. Carbodiimides (-N=C=N-)O. Isothiocyanates (-N=C=S)P. Nitriles (-C≡N)1. Aliphatic2. Olefinic3. AromaticQ. Cyanamides (=N-C≡N)R. Isocyanides (-N≡C )S. Thiocyanates (-S-C≡N)T. Nitroso Compounds (-N=O)U. N-Nitroso Compounds (=N-N=O)V. Nitrates (-O-NO2)W. Nitrites (-O-N=O)X. Nitro Compounds (-NO2)1. Aliphatic2. AromaticY. N-Nitro-Compounds (=N-NO2)IV. Silicon Containing Compounds (Except Si-O)V. Phosphorus Containing Compounds (Except P-O and P(=O)-O) VI. Sulfur Containing CompoundsA. Sulfides (R-S-R)1. Aliphatic2. AromaticB. Disulfides (R-S-S-R)C. Thiols1. Aliphatic2. AromaticD. Sulfoxides (R-S(=O)-R)E. Sulfones (R-SO2-R)F. Sulfonyl Halides (R-SO2-X)G. Sulfonic Acids (R-SO2-OH)1. Sulfonic Acid Salts (R-SO2-O-M)2. Sulfonic Acid Esters (R-SO2-O-R)3. Sulfuric Acid Esters (R-O-S(=O)-O-R)4. Sulfuric Acid Salts (R-O-SO2-O-M)H. Thioamides (R-C(=S)-NH2)I. Thioureas (R-NH-C(=S)-NH2)J. Sulfonamides (R-SO2-NH2)VII. Oxygen Containing Compounds (Except -C(=O)-)A. Ethers1. Aliphatic Ethers (R-O-R)2. Alicyclic Ethers3. Aromatic Ethers4. Furans5. Silicon Ethers (R3-Si-O-R)6. Phosphorus Ethers ((R-O)3-P)B. Alcohols (R-OH)1. Primarya. Aliphaticb. Olefinicc. Aromatic2. Secondarya. Aliphaticb. Aromatic3. Tertiarya. Aliphaticb. Aromatic4. Diols and Polyols5. Carbohydrates6. PhenolsVIII. Compounds Containing Carbon To Oxygen Double BondsA. Ketones (R-C(=O)-R)1. Aliphatic and Alicyclic2. Olefinic3. Aromatic4. a-Diketones and b-DiketonesB. Aldehydes (R-C(=O)-H)C. Acid Halides (R-C(=O)-X)D. Anhydrides (R-C(=O)-O-C(=O)-R)E. Amides1. Primary (R-C(=O)-NH2)2. Secondary (R-C(=O)-NH-R)3. Tertiary (R-C(=O)-N-R2)F. Imides (R-C(=O)-NH-C(=O)-R)G. Hydrazides (R-C(=O)-NH-NH2)H. Ureas (R-NH-C(=O)-NH2)I. Hydantoins, Uracils, BarbituratesJ. Carboxylic Acids (R-C(=O)-OH)1. Aliphatic and Alicyclic2. Olefinic3. Aromatic4. Amino Acids5. Salts of Carboxylic AcidsK. Esters1. Aliphatic Esters of Aliphatic Acids2. Olefinic Esters of Aliphatic Acids3. Aromatic Esters of Aliphatic Acids4. Cyclic Esters (Lactones)5. Chloroformates6. Carbamates7. Esters of Phosphorus AcidsPublished by Bio-Rad Laboratories, Inc., Informatics Division. © 1978-2004 Bio-Rad Laboratories, Inc. All Rights Reserved.Go to: home • ir • proton nmr • carbon nmr• mass specTable of Contents - Carbon NMRI. HydrocarbonsII. Halogenated HydrocarbonsIII. Nitrogen Containing CompoundsIV. Silicon Containing Compounds (Except Si-O)V. Phosphorus Containing Compounds (Except P-O And P(=O)-O) VI. Sulfur Containing CompoundsVII. Oxygen Containing Compounds (Except -C(=O)-)VIII. Compounds Containing Carbon To Oxygen Double BondsI. HydrocarbonsA. Saturated Hydrocarbons1. Normal Alkanes2. Branched Alkanes3. Cyclic AlkanesB. Unsaturated Hydrocarbons1. Acyclic Alkenes2. AlkynesC. Aromatic Hydrocarbons1. Monocyclic (Benzenes) and PolycyclicII. Halogenated HydrocarbonsA. Fluorinated Hydrocarbons1. Aliphatic2. AromaticB. Chlorinated Hydrocarbons1. Aliphatic2. AromaticC. Brominated Hydrocarbons1. Aliphatic2. AromaticD. Iodinated Hydrocarbons1. Aliphatic2. AromaticIII. Nitrogen Containing CompoundsA. Amines1. Primarya. Aliphaticb. Aromatic2. Secondarya. Aliphaticb. Aromatic3. Tertiarya. Aliphaticb. AromaticB. PyridinesC. Amine SaltsD. Oximes (-CH=N-OH)E. Quaternary Ammonium SaltsF. Nitriles (-C≡N)1. Aliphatic2. Olefinic3. AromaticG. Thiocyanates (-S-C≡N)H. Nitro Compounds (-NO2)1. Aliphatic2. AromaticIV. Silicon Containing Compounds (Except Si-O)V. Phosphorus Containing Compounds (Except P-O and P(=O)-O) VI. Sulfur Containing CompoundsA. Sulfides (R-S-R)1. Aliphatic2. AromaticB. Disulfides (R-S-S-R)C. Thiols1. Aliphatic2. AromaticD. Sulfones (R-SO2-R)VII. Oxygen Containing Compounds (Except -C(=O)-)A. Ethers1. Aliphatic Ethers (R-O-R)2. Alicyclic Ethers3. Aromatic EthersB. Alcohols (R-OH)1. Primarya. Aliphatic and Alicyclicb. Aromatic2. Secondarya. Aliphatic and Alicyclic3. Tertiarya. Aliphatic4. PhenolsVIII. Compounds Containing Carbon To Oxygen Double BondsA. Ketones (R-C(=O)-R)1. Aliphatic and Alicyclic2. AromaticB. Aldehydes (R-C(=O)-H)C. Acid Halides (R-C(=O)-X)D. Anhydrides (R-C(=O)-O-C(=O)-R)E. Amides1. Primary (R-C(=O)-NH2)2. Secondary (R-C(=O)-NH-R)3. Tertiary (R-C(=O)-N-R2)F. Carboxylic Acids (R-C(=O)-OH)1. Aliphatic and Alicyclic2. AromaticG. Esters1. Aliphatic Esters of Aliphatic Acids2. Olefinic Esters of Aliphatic Acids3. Aromatic Esters of Aliphatic AcidsPublished by Bio-Rad Laboratories, Inc., Informatics Division. © 1978-2004 Bio-Rad Laboratories, Inc. All Rights Reserved.Go to: home • ir • proton nmr • carbon nmr• mass specTable of Contents - MSComing SoonI. HydrocarbonsII. Halogenated HydrocarbonsIII. Nitrogen Containing CompoundsIV. Silicon Containing Compounds (Except Si-O)V. Phosphorus Containing Compounds (Except P-O And P(=O)-O) VI. Sulfur Containing CompoundsVII. Oxygen Containing Compounds (Except -C(=O)-)VIII. Compounds Containing Carbon To Oxygen Double BondsI. HydrocarbonsA. Saturated Hydrocarbons1. Normal Alkanes2. Branched Alkanes3. Cyclic AlkanesB. Unsaturated Hydrocarbons1. Acyclic Alkenes2. Cyclic Alkenes3. AlkynesC. Aromatic Hydrocarbons1. Monocyclic (Benzenes)2. PolycyclicII. Halogenated HydrocarbonsA. Fluorinated Hydrocarbons1. Aliphatic2. AromaticB. Chlorinated Hydrocarbons1. Aliphatic2. Olefinic3. AromaticC. Brominated Hydrocarbons1. Aliphatic2. Olefinic3. AromaticD. Iodinated Hydrocarbons1. Aliphatic and Olefinic2. AromaticIII. Nitrogen Containing CompoundsA. Amines1. Primarya. Aliphatic and Olefinicb. Aromatic2. Secondarya. Aliphatic and Olefinicb. Aromatic3. Tertiarya. Aliphatic and Olefinicb. AromaticB. PyridinesC. QuinolinesD. Miscellaneous Nitrogen HeteroaromaticsE. HydrazinesF. Amine SaltsG. Oximes (-CH=N-OH)H. Hydrazones (-CH=N-NH2)I. Azines (-CH=N-N=CH-)J. Amidines (-N=CH-N)K. Hydroxamic AcidsL. Azo Compounds (-N=N-)M. Triazenes (-N=N-NH-)N. Isocyanates (-N=C=O)O. Carbodiimides (-N=C=N-)P. Isothiocyanates (-N=C=S)Q. Nitriles (-C≡N)1. Aliphatic2. Olefinic3. AromaticR. Cyanamides (=N-C≡N)S. Thiocyanates (-S-C≡N)T. Nitroso Compounds (-N=O)U. N-Nitroso Compounds (=N-N=O)V. Nitrites (-O-N=O)W. Nitro Compounds (-NO2)1. Aliphatic2. AromaticX. N-Nitro-Compounds (=N-NO2)IV. Silicon Containing Compounds (Except Si-O)V. Phosphorus Containing Compounds (Except P-O and P(=O)-O) VI. Sulfur Containing CompoundsA. Sulfides (R-S-R)1. Aliphatic2. Heterocyclic3. AromaticB. Disulfides (R-S-S-R)C. Thiols1. Aliphatic2. AromaticD. Sulfoxides (R-S(=O)-R)E. Sulfones (R-SO2-R)F. Sulfonyl Halides (R-SO2-X)G. Sulfonic Acids (R-SO2-OH)1. Sulfonic Acid Salts (R-SO2-O-M)2. Sulfonic Acid Esters (R-SO2-O-R)3. Sulfuric Acid Esters (R-O-S(=O)-O-R)H. Thioamides (R-C(=S)-NH2)I. Thioureas (R-NH-C(=S)-NH2)J. Sulfonamides (R-SO2-NH2)K. Sulfamides (R-NH-SO2-NH-R)VII. Oxygen Containing Compounds (Except -C(=O)-)A. Ethers1. Aliphatic Ethers (R-O-R)2. Acetals (R-CH-(-O-R)2)3. Alicyclic Ethers4. Aromatic Ethers5. Furans6. Silicon Ethers (R3-Si-O-R)7. Phosphorus Ethers ((R-O)3-P)8. Peroxides (R-O-O-R)B. Alcohols (R-OH)1. Primarya. Aliphatic and Alicyclicb. Olefinicc. Aromaticd. Heterocyclic2. Secondarya. Aliphatic and Alicyclicb. Olefinicc. Aromatic3. Tertiarya. Aliphaticb. Olefinicc. Aromatic4. Diols5. Carbohydrates6. PhenolsVIII. Compounds Containing Carbon To Oxygen Double BondsA. Ketones (R-C(=O)-R)1. Aliphatic and Alicyclic2. Olefinic3. Aromatic4. α-Diketones and β-DiketonesB. Aldehydes (R-C(=O)-H)C. Acid Halides (R-C(=O)-X)D. Anhydrides (R-C(=O)-O-C(=O)-R)E. Amides1. Primary (R-C(=O)-NH2)2. Secondary (R-C(=O)-NH-R)3. Tertiary (R-C(=O)-N-R2)F. Imides (R-C(=O)-NH-C(=O)-R)G. Hydrazides (R-C(=O)-NH-NH2)H. Ureas (R-NH-C(=O)-NH2)I. Hydantoins, Uracils, BarbituratesJ. Carboxylic Acids (R-C(=O)-OH)1. Aliphatic and Alicyclic2. Olefinic3. Aromatic4. Amino Acids5. Salts of Carboxylic AcidsK. Esters1. Aliphatic Esters of Aliphatic Acids2. Olefinic Esters of Aliphatic Acids3. Aliphatic Esters of Olefinic Acids4. Aromatic Esters of Aliphatic Acids5. Esters of Aromatic Acids6. Cyclic Esters (Lactones)7. Chloroformates8. Esters of Thio-Acids9. Carbamates10. Esters of Phosphorus AcidsPublished by Bio-Rad Laboratories, Inc., Informatics Division. © 1978-2004 Bio-Rad Laboratories, Inc. All Rights Reserved.Go to: home • ir • proton nmr • carbon nmr• mass specSaturated HydrocarbonsNormal Alkanes1. C-H stretching vibration:CH3 asymmetric stretching, 2972-2952 cm-1CH3 symmetric stretching, 2882-2862 cm-1CH2 asymmetric stretching, 2936-2916 cm-1CH2 symmetric stretching, 2863-2843 cm-12. C-H bending vibration:CH3 asymmetric bending, 1470-1430 cm-1CH2 asymmetric bending, 1485-1445 cm-1(overlaps band due to CH3 asymmetricbending)3. C-H bending vibration:CH3 symmetric bending, 1380-1365 cm-1(when CH3 is attached to a C atom)4. C-H wagging vibration:CH2 out-of-plane deformations wagging, 1307-1303 cm-1 (weak) 5. CH2 rocking vibration:(CH2)2 in-plane deformations rocking, 750-740 cm-1(CH2)3 in-plane deformations rocking, 740-730 cm-1(CH2)4 in-plane deformations rocking, 730-725 cm-1(CH2) ≥ 6 in-plane deformations rocking, 722 cm-1Splitting of the absorption band occurs in most cases (730 and 720 cm-1) when the long carbon-chain alkane is in the crystalline state (orthorombic or monoclinic form).Coming Soon!Click on a vibrational mode link in the table to the leftor the spectrum above to visualize the vibrational mode here.Published by Bio-Rad Laboratories, Inc., Informatics Division. © 1978-2004 Bio-Rad Laboratories, Inc. All Rights Reserved.Saturated HydrocarbonsBranched Alkanes1. C-H stretching vibration:CH3 asymmetric stretching, 2972-2952 cm-1CH3 symmetric stretching, 2882-2862 cm-1CH2 asymmetric stretching, 2936-2916 cm-1CH2 symmetric stretching, 2863-2843 cm-12. C-H bending vibration:CH3 asymmetric bending, 1470-1430 cm-1CH2 asymmetric bending, 1485-1445 cm-1(overlaps band due to CH3 symmetric bending)3. C-H bending vibration:-C-C(CH3)-C-C- symmetric bending, 1380-1365 cm-1(when CH3 is attached to a C atom)-C-C(CH3)-C(CH3)-C-C- symmetric bending, 1380-1365 cm-1(when CH3 is attached to a C atom)(CH3)2CH- symmetric bending, 1385-1380 cm-1and 1365 cm-1(two bands of about equal intensity)-C-C(CH3)2-C- symmetric bending,1385-1380 cm-1and 1365 cm-1 (two bands of about equal intensity).(CH3)3C- symmetric bending, 1395-1385 cm-1and 1365 cm-1(two bands of unequal intensity with the 1365 cm-1 band as the much stronger component of the doublet).4. Skeletal vibration:-C-C(CH3)-C-C-,1159-1151cm-1-C-C(CH3)-C(CH3)-C-C-,1130-1116 cm-1(CH3)CH-,1175-1165 cm-1 and 1170-1140 cm-1-C-C(CH3)2-C-,1192-1185 cm-1(CH3)3C-, 1255-1245 cm-1 and 1250-1200 cm-15. C-H rocking vibration:(CH2)2 in-plane deformations rocking, 750-740 cm-1(CH2)3 in-plane deformations rocking, 740-730 cm-1(CH2)4 in-plane deformations rocking, 730-725 cm-1(CH2) ≥ 6 in-plane deformations rocking, 722 cm-1Coming Soon!Click on a vibrational mode link in the table to the left or the spectrum above to visualize the vibrational modehere.Published by Bio-Rad Laboratories, Inc., Informatics Division. © 1978-2004 Bio-Rad Laboratories, Inc. All Rights Reserved.Saturated Hydrocarbons Cyclic AlkanesCyclopropanes1. C-H stretching vibration:ring CH 2 asymmetric stretching, 3100-3072 cm -1 ring CH 2 symmetric stretching, 3030-2995 cm -12. Ring deformation vibration:ring deformation, 1050-1000 cm -13. C-H deformation vibration: CH 2 wagging, 860-790 cm -1Cyclobutanes1. C-H stretching vibration:ring CH 2 asymmetric stretching, 3000-2974 cm -1 ring CH 2 symmetric stretching, 2925-2875 cm -12. C-H deformation vibration:ring CH 2 asymmetric bending, ca 1444 cm -13. Ring deformation vibration:ring deformation, 1000-960 cm -1 888-838 cm -14. C-H deformation vibration:ring CH 2 rocking, 950-900 cm -1Cyclopentanes1. C-H stretching vibration:ring CH 2 asymmetric stretching, 2960-2952 cm -1 ring CH 2 symmetric stretching, 2866-2853 cm -1 2. C-H deformation vibration:ring CH 2 asymmetric bending, ca 1455 cm -1 3. Ring deformation vibration:ring deformation, 1000-960 cm -1 4. C-H deformation vibration:ring CH 2rocking, 930-890 cm -1Cyclohexanes1. C-H stretching vibration:ring CH 2 asymmetric stretching, ca 2927 cm -1ring CH 2 symmetric stretching, ca 2854 cm -1 2. C-H deformation vibration:ring CH 2 asymmetric bending, ca 1462 cm -1 3. C-H deformation vibration:ring CH 2 wagging, ca 1260 cm -1 4. Ring deformation vibration:ring deformation, 1055-1000 cm -1 1000- 952 cm -1 5. C-H deformation vibration:ring CH 2 rocking, 890-860 cm -16. The spectra of cyclic alkanes of five or more ring carbons show ring CH 2 stretching frequencies which overlap those of CH 3 and CH 2 groups of their alkyl substituents. These frequencies also overlap thoseof the CH 3 and CH 2 stretching frequencies of acylic alkanes. When samples of unknown composition are examined for the presence of such ring structures, the absorption bands of their spectra at the C-H stretching region should havethe best possible resolution.Coming Soon!Click on a vibrational mode link in the table to the left or the spectrum above to visualize the vibrational modehere.Numerous references cite the spectral region of 2800-2600 cm-1 for obtainingconfirmatory evidence of the presence of saturated simple ring structures. Absorptionat this region consists of a weak band or bands whose pattern and band locations arehelpful in confirming or indicating the presence of these rings. Although such absorptionfeatures have a limited diagnostic value, it is most reliable when the absorption occursin the spectra of simple saturated aliphatic hydrocarbons.Cycloalkanes (8, 9, and 10 C atoms)1 C-H stretching vibration:ring CH2 asymmetric stretching, ca 2930 cm-1ring CH2 symmetric stretching, ca 2850 cm-12. C-H deformation vibration:ring CH2 asymmetric bending, 2 or 3 absorption bands,1487-1443 cm-1Published by Bio-Rad Laboratories, Inc., Informatics Division. © 1978-2004 Bio-Rad Laboratories, Inc. All Rights Reserved.Go to: home • ir • proton nmr • carbon nmr• mass specUnsaturated HydrocarbonsAcyclic AlkenesMonosubstituted Alkenes (vinyl)1. C=C stretching vibration:C=C stretching, 1648-1638 cm-12. C-H deformation vibration:trans CH wagging, 995-985 cm-1CH2 wagging, 910-905 cm-13. C-H stretching vibration:CH2 asymmetric stretching, 3092-3077 cm-1CH2 symmetric stretching and CH stretching, 3025-3012 cm-1 4. C-H deformation vibration:CH2 asymmetric bending, 1420-1412 cm-15. C-H deformation vibration overtone:overtone of CH2 wagging, 1840-1805 cm-1Asymmetric Disubstituted Alkenes (vinylidine)1. C=C stretching vibration:C=C stretching, 1661-1639 cm-12. C-H deformation vibration:CH2 wagging, 895-885 cm-13. C-H stretching vibration:CH2 stretching asymmetric, 3100-3077 cm-14. C-H deformation vibration overtone:overtone of CH2 wagging, 1792- 1775 cm-1Symmetric Disubstituted Alkenes (cis)1. C=C stretching vibration:C=C stretching, 1662- 1631 cm-12. C-H deformation vibration:cis CH wagging, 730- 650 cm-13. C-H stretching vibration:CH stretching, 3050-3000 cm-1Symmetric Disubstituted Alkenes (trans)1. C=C stretching vibration:C=C stretching, ca 1673 cm-1, very weak or absent2. C-H deformation vibration:trans CH wagging, 980-965 cm-13. C-H stretching vibration:CH stretching, 3050-3000 cm-1Trisubstituted Alkenes1. C=C stretching vibration:C=C stretching, 1692-1667 cm-12. C—H deformation vibration:C-H wagging, 840-790 cm-13. C-H stretching vibration:C-H stretching, 3050-2990 cm-1Coming Soon!Click on a vibrational mode link in the table to the left or the spectrum above to visualize the vibrational modehere.Tetrasubstituted Alkenes1. C=C stretching vibration:C=C stretching, 1680-1665 cm-1, very weak or absentNOTES: The C=C stretching vibration of molecules which maintain acenter of symmetry absorbs very weakly, if at all, in the infrared region and,usually, is difficult to detect. This is true of the trans isomers and thetetrasubstitutedC=C linkages.When two or more olefinic groups occur in the hydrocarbon molecule, the infraredabsorption spectrum shows the additive and combined absorption of theunsaturatedgroups. However, if the unsaturated groups are subject to conjugation, the C=Cstretchingfrequency, usually, is lowered and a splitting of the C=C stretching frequencyband occurs.Conjugation also intensifies the C=C stretching frequency of trans unsaturatedgroups.Published by Bio-Rad Laboratories, Inc., Informatics Division. © 1978-2004 Bio-Rad Laboratories, Inc. All Rights Reserved.Go to: home • ir • proton nmr • carbon nmr• mass specUnsaturated Hydrocarbons Cyclic AlkenesEndocyclic C=CEndocyclic C=C corresponds to cis symmetrically disubstituted C=C of acyclic alkenes.1. C=C stretching, vibration:C=C stretching, near 1650 cm -1(except cyclobutene, 1560 cm -1 and cyclopentene, 1611 cm -1)2. C-H deformation vibration: CH wagging, 730- 650 cm -13. C-H stretching vibration:CH stretching, 3075- 3010 cm -1(usually two bands, asymmetric stretching and symmetric stretching for 4, 6, 7, and 8 membered rings)1- substituted endocyclic C=C1- substituted endocyclic C=C corresponds to trisubstituted acyclic alkenes.1. C=C stretching vibration:C=C stretching, near 1650 cm -1 (frequency raised)2. C-H deformation vibration: CH wagging, 840-790 cm -13. C-H stretching vibration:CH stretching, near 3000 cm -11.2- disubstituted endocyclic C=C1. C=C stretching vibration:C=C stretching, 1690-1670 cm -1 (4, 5, and 6 membered rings)Exocyclic C=CH 2Exocyclic C=CH 2 corresponds to the asymmetrically disubstituted C=C of acyclic alkenes (vinylidine).1. C=C stretching,1678-1650 cm -1 (4, 5, and 6 membered rings)2. C-H deformation vibration:=CH 2 wagging, 895-885 cm -13. C-H stretching vibration:=CH 2 stretching, near 3050 cm -1NOTES: The C=C stretching frequency of both the endocyclic HC=CH and the exocyclic C=CH 2 is sensitive to ring strain. As the ring size decreases from 6 to 4 members, the C=C stretching frequency of the endocyclic HC=CH is lowered. However, for the C=C stretching frequency of exocyclic C=CH 2, a gradual increase in the C=C stretching frequency occurs as the ring gets smaller. Substitution of methyl groups for the hydrogens of the endocyclic HC=CH and the exocyclic C=CH 2 cause an increase in the C=C stretching frequency.When two or more C=C groups occur in the hydrocarbon molecule, the infrared absorption spectrum shows the additive and combined absorption effects of the unsaturated groups. If such groups are subject to conjugation, the C=C stretching frequency is lowered and asplitting of the C=C stretching frequency band occurs.Coming Soon!Click on a vibrational mode link in the table to the left or the spectrum above to visualize the vibrational modehere.Published by Bio-Rad Laboratories, Inc., Informatics Division. © 1978-2004 Bio-Rad Laboratories, Inc. All Rights Reserved.Unsaturated Hydrocarbons AlkynesMonosubstituted Alkynes (RC ≡CH)1. C ≡C stretching vibration:C ≡C stretching, 2140-2100 cm -12. C-H stretching vibration:≡CH bending, ca 3300 cm -13. C-H deformation vibration: ≡CH bending, 642-615 cm -14. C-H deformation vibration overtone:overtone of ≡CH deformation, 1260-1245 cm -1Disubstituted Alkynes (RC ≡CR')1. C ≡C stretching vibration:C ≡C stretching, 2260-2190 cm -1 (unconjugated)NOTES: Although the intensity of the absorption band caused bythe C ≡C stretching vibration is variable, it is strongest when the alkyne group is monosubstituted. When this group is disubstituted in open chain compounds, the intensity of the C ≡C stretching vibration band diminishesas its position in the molecule tends to establish a pseudo center of symmetry. In some instances this band is too weak to be detected and, thus, its absence in the spectrum does not, necessarily, establish proof of the absence of this linkage.Occasionally, the spectra of disubstituted alkynes show two or more bands at the C ≡C stretching region.Conjugation with olefinic double bonds or aromatic rings tend to slightly increase the intensity of the C ≡C stretching vibration band and shift it toa lower frequency.Coming Soon!Click on a vibrational mode link in the table to the left or the spectrum above to visualize the vibrational modehere.Published by Bio-Rad Laboratories, Inc., Informatics Division . © 1978-2004 Bio-Rad Laboratories, Inc. All Rights Reserved.。
有机化学术语(中英文对照)
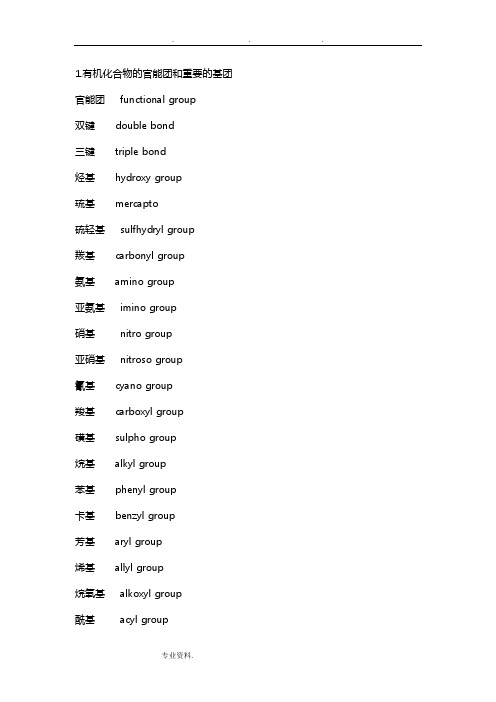
1.有机化合物的官能团和重要的基团官能团functional group双键double bond三键triple bond烃基hydroxy group琉基mercapto硫轻基sulfhydryl group羰基carbonyl group氨基amino group亚氨基imino group硝基nitro group亚硝基nitroso group氰基cyano group羧基carboxyl group磺基sulpho group烷基alkyl group苯基phenyl group卡基benzyl group芳基aryl group烯基allyl group烷氧基alkoxyl group酰基acyl group活性亚甲基active methylene group2.有机化合物的类型烃hydrocarbon石蜡paraffin脂肪烃aliphatic hydrocarbon烷烃alkane烯烃alkene炔烃alkyne共扼二烯烃conjugated diene脂环烃alicyclic hydrocarbon螺环化合物spiro compound桥环化合物bridged ring compound芳烃aromatic hydrocarbon非苯芳烃nonbenzenoid aromatic hydrocarbon 稠环芳烃condensed aromatics卤代烃halohydrocarbon醇alcohol酚phenol醚ether环氧化合物epoxide冠醚crown ether硫醇thiol硫酚thiophenol硫醚sulfide二硫化物disulfide亚磺酸sulfinic acid磺酸sulfonic acid 亚砜sulfoxide砜sulfone醛aldehyde酮ketone半缩醛hemiacetaI半缩酮hemiketal缩醛acetal缩酮ketal西佛碱shiff's base肟oxime腙hydrozone缩氨脲semicarbazoneα,β-不饱和酮α,β--unsaturated ketone 醌quinone羧酸carboxylic acid酰卤acid halide酸酐acid anhydride酯ester酰胺amide內酯lactone内酰胺lactam月青nitrile取代酸substituted acid羟基酸hydroxy acid醇酸alcoholic acid酚酸phenolic acid酮酸keto acidB-酮酸酯B-ketone ester乙酰乙酸乙醋ethyl acetoacetate亚硝基化合物nitroso compound硝基化合物njtro compound亚胺imine胺amine伯胺primary amine仲胺secondary amine叔胺tertiary amine季铵盐quaternary ammonium salt季铵碱quaternary ammonium hydroxide 重氮盐diazonium salt偶氮化合物azo compound胍guanidine氨基酸amino acid磷phosphine磷酸酯phosphate亚磷酸酯phosphite膦酸酯phosphonate膦酸phosphonic acid3.杂环化合物吡咯pyrrol呋喃furane噻吩thiophone吲哚indole卟吩porphine咪唑imidazole噻唑thioazole吡啶pyridine喹啉quinoline异喹啉isoquinoline吡喃鎓盐pyrylium salts 黄酮flavone嘧啶pirimidine嘌呤purine4.有机天然产物肽peptide多肽polypeptide核酸nucleic acid核苷nucleoside核苷酸nucleotide生物碱alkaloid碳水化合物carbohydrate单糖monosaccharide醛糖aldoses酮糖ketosesD-核糖ribose D-2-脱氧核糖deoxyribose 葡萄糖glucose果糖fructose糖脎osazone糖苷glucoside低聚糖oligosaccharide 麦芽糖maltose蔗糖sucrose纤维二糖cellobiose环糊精cyclodextrin多糖polysaccharide淀粉starch纤维素cellulose类脂lipid萜类化合物terpenoid甾族化合物steroid脂肪fat油oil脂肪酸fatty acid甘油三羧酸酯triglyceride磷脂phospholipid磷脂酸phosphalidic acid蜡wax5.有机化合物的结构理论价键理论valence-bond theory分子轨道理论molecular orbital theory 共振论resonance theory凯库勒式Kekule formula路易斯式Lewis formulaσ键σ bondπ键π bond键能bond energy键角bond angle键长bond Iength成键轨道bonding orbital反键轨道antibonding orbital最高已占轨道HOMO highest occupied molecular orbital 最低末占轨道LUMO lowest unoccupied molecular orbital 诱导效应inductive effect共轭效应conjugated effectπ,π-共轭π,π- conjugationp,π-共轭p,π- conjugation超共轭作用hyperconjugation离域能delocalization energy共振能resonance energy给电子基团electron donating group吸电子基团electron withdrawing group芳性aromaticity休克尔规律Huckel's rule两性离子Zwitterion6.有机化学中的同分异构异构体isomer构造constitution构型configuration构象conformation构造异构constitutional isomerism立体异构stereo isomerism构型异构configurational isomerism顺反异构cis-trans isomerism次序规则sequence ruIe同侧Zugammen Z异侧Entgegen E顺式cis反式trans对映异构enantiomerism = 光学异构旋光异构optical isomerism旋光性optical activity旋光度optical rotation比旋光度specific rotation对称面plane of symmetry对称中心center of symmetry对称轴axis of symmetry手性chirality手性分子chiral molecules对映异构体,对映体enantiomer 非对映体diastereomer外消旋体raceme左旋体leveisomer右旋体dextroisomer内消旋体mesomer费歇尔投影式Fischer projection相对构型relative configuration绝对构型absolute configurationR -构型R -configurationS -构型S -configuration赤式erythro苏式threo外消旋化racemization拆分resolution光学纯度Optical Purity对映体过量百分数enantiomeric excess立体专一性反应stereospecific reaction 立体选择性反应stereoselective reaction不对称合成asymmetric synthesis构象异构conformational isomerism构象分析conformational analysis锯架式perspective formula 纽曼投影式Newman projection formula椅式chair form船式boat form直立键a键axial bond 平伏键e键equatorial bond互变异构tautomerism酮式keto-form烯醇式enol-form差向异构化epimerization变旋现象mutamerism哈武斯式Haworth form7.有机反应的名称取代反应substitution reaction加成反应addition reaction马尔科夫尼可夫规律Markovnikov rule 共轭加成conjugate addition消去反应elemination reaction查依采夫规律Saytzeff rule霍夫曼规律Hofmann rule硼氢化反应hydroboration催化加氢catalytic hydrogenation 聚合反应polymerization单体monomer聚合物polymer硝化反应nitration卤化反应halogenation磺化反应sulfonation烷基化反应alkylation酰基化反应acylation酯化反应esterification酯交换反应transesterification脱羧反应decarboxylation 氯甲基化反应chloromethylation傅列德尔-克拉夫茨反应Friedel-Crafts reaction格利雅反应Grignard reaction 格利雅试剂(格氏试剂) Grignard reagent赖默-梯曼反应Reimer-Tiemann reaction 卤仿反应haloform reaction水解反应hydrolysis reaction醇解反应alcoholysis reaction氨解反应ammonolysisi reaction皂化saponification插烯作用vinylogy缩合condensation克莱森缩合Claisen condensation安息香缩合benzoin condensation羟醛缩合aldol condensation列弗尔马茨基反应Reformatsky reaction迈克尔反应Michael reaction诺文格尔反应Knoevenagel reaction加布里反应Gabriel reaction乙酰乙酸乙酯合成法acetoacetic ester synthesis 丙二酸酯合成法malonic ester synthesis 威廉逊合成法William Son synthesis海森堡试验Hinsberg test重氮化反应diazotization reaction偶联反应coupling reaction脱氨基反应deamination reaction维悌希反应Wittig reaction氧化反应oxidation reaction还原反应reduction reaction周环反应pericyclic reaction环加成反应cycloaddition reaction电环化反应electrocyclic reaction坎尼扎罗反应Cannizzaro reaction齐齐巴宾反应Chichibabin reaction狄尔斯-阿德尔反应Diels-alder reaction斐林试剂Fehling reagent托伦试剂Tollens reagent沃克还原Wolff-Kishner reduction罗森蒙德还原Rosenmund reduction克莱门森还原Clemmenson reduction考普重排Cope rearrangement霍夫曼重排Hofmann rearrangement嚬哪醇重排pinacol rearrangement弗里茨重排Fries rearrangement克莱森重排Claisen rearrangement二烯体diene亲二烯体dienophile分子轨道对称守恒原理conversation of orbital symmetry8.有机反应机理均裂homolytic异裂heterolytic活性中间体active intermediate碳正离子carbocation碳负离子carbanion烯醇负离子enolate anion自由基,游离基free radical卡宾,碳烯carbene氮烯nitrene速度决定步骤rate-determining step哈蒙特假定Hammond postulate能线图energy profile过渡状态transition state邻基参与neighboring group participation动力学控制kinetic control热力学控制thermodynamic control离去基团leaving group底物substrate亲电试剂electrofphile亲核试剂nucleophile亲电加成反应electrophilic addition亲电取代反应electrophilic substitution定位规律orientation rule亲核取代反应nucleophilic substitutionSN2 反应机理SN2 reaction mechanismSN1 反应机理SN1 reaction mechanism瓦尔登转化Walden inversion亲核加成反应nucleophilic addition亲核加成-消去反应nucleophilic addition-elimination reaction 消去反应机理elimination reaction mechanismE1 反应机理E1 reaction mechanismE2 反应机理E2 reaction mechanism反式消去anti elimination重排反应机理rearrangement reaction mechanism 自由基反应free radical reaction链引发chain initation链增长chain propagation链终止chain termination9.有机化合物的光谱红外光谱IR Infrared spectra傅立叶变换Fourier Transform指纹区finger print region吸收频率absorption frequency紫外光谱UV Ultraviolet spectra电子跃迁elctronic transition吸光度absorbance摩尔消光系数molar extinction coefficient发色团chromophore助色团auxochrome核磁共振NMR Nuclear Magnetic Resonance1HNMR 谱1HNMR spectra13CNMR 谱13CNMR spectra屏蔽效应shielding effect化学位移chemical shift自旋偶合spin-spin coupling自旋裂分spin-spin splitting偶合常数coupling constant质子去偶proton spin decoupling 质子偏共振去偶proton off-resonance decoupling质谱Mass Spectra(MS)电子流轰击election impact (EI)快原子轰击fast atom bombarment (FAB)分子离子峰molecular ion peak同位素峰isotopic peak基峰base peak质荷比(m/z) mass-to-charge ratio10.分子间作用力氢键hydrogen bond色散力dispersion force 范德华力Van Der Waals force 偶极-偶极作用力dipole-dipole interraction force11.物理性质熔点melting point沸点boiling point密度density溶解度solubility偶极矩dipole moment12.有机化合物的酸碱性酸性acidity碱性basicity<HTML>本站材料仅为本院教师与学生教学所用,请勿它用!</HTML>有机化合物编辑[yǒu jīhuàhéwù]有机化合物主要由氢元素、碳元素组成,含碳的化合物,但是不包括一氧化碳、二氧化碳和以碳酸根结尾的物质。
铑催化剂催化烯烃不对称加氢反应研究进展
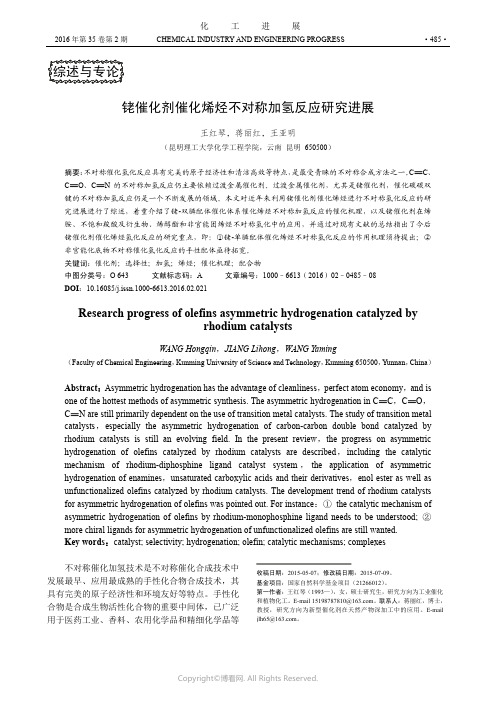
2016年第35卷第2期CHEMICAL INDUSTRY AND ENGINEERING PROGRESS ·485·化工进展铑催化剂催化烯烃不对称加氢反应研究进展王红琴,蒋丽红,王亚明(昆明理工大学化学工程学院,云南昆明 650500)摘要:不对称催化氢化反应具有完美的原子经济性和清洁高效等特点,是最受青睐的不对称合成方法之一。
C=C、C=O、C=N的不对称加氢反应仍主要依赖过渡金属催化剂。
过渡金属催化剂,尤其是铑催化剂,催化碳碳双键的不对称加氢反应仍是一个不断发展的领域。
本文对近年来利用铑催化剂催化烯烃进行不对称氢化反应的研究进展进行了综述,着重介绍了铑-双膦配体催化体系催化烯烃不对称加氢反应的催化机理,以及铑催化剂在烯胺、不饱和羧酸及衍生物、烯醇酯和非官能团烯烃不对称氢化中的应用,并通过对现有文献的总结指出了今后铑催化剂催化烯烃氢化反应的研究重点,即:①铑-单膦配体催化烯烃不对称氢化反应的作用机理须待提出;②非官能化底物不对称催化氢化反应的手性配体亟待拓宽。
关键词:催化剂;选择性;加氢;烯烃;催化机理;配合物中图分类号:O 643 文献标志码:A 文章编号:1000–6613(2016)02–0485–08DOI:10.16085/j.issn.1000-6613.2016.02.021Research progress of olefins asymmetric hydrogenation catalyzed byrhodium catalystsWANG Hongqin,JIANG Lihong,WANG Yaming(Faculty of Chemical Engineering,Kunming University of Science and Technology,Kunming 650500,Yunnan,China)Abstract:Asymmetric hydrogenation has the advantage of cleanliness,perfect atom economy,and is one of the hottest methods of asymmetric synthesis. The asymmetric hydrogenation in C=C,C=O,C=N are still primarily dependent on the use of transition metal catalysts. The study of transition metal catalysts,especially the asymmetric hydrogenation of carbon-carbon double bond catalyzed by rhodium catalysts is still an evolving field. In the present review,the progress on asymmetric hydrogenation of olefins catalyzed by rhodium catalysts are described,including the catalytic mechanism of rhodium-diphosphine ligand catalyst system,the application of asymmetric hydrogenation of enamines,unsaturated carboxylic acids and their derivatives,enol ester as well as unfunctionalized olefins catalyzed by rhodium catalysts. The development trend of rhodium catalysts for asymmetric hydrogenation of olefins was pointed out. For instance:①the catalytic mechanism of asymmetric hydrogenation of olefins by rhodium-monophosphine ligand needs to be understood; ② more chiral ligands for asymmetric hydrogenation of unfunctionalized olefins are still wanted.Key words:catalyst; selectivity; hydrogenation; olefin; catalytic mechanisms; complexes不对称催化加氢技术是不对称催化合成技术中发展最早、应用最成熟的手性化合物合成技术,其具有完美的原子经济性和环境友好等特点。
PEM电解水析氧催化剂研究进展
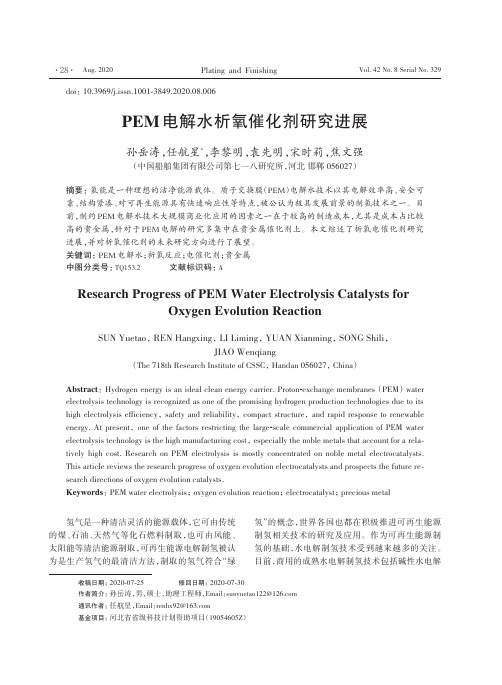
PEM电解水析氧催化剂研究进展孙岳涛,任航星∗,李黎明,袁先明,宋时莉,焦文强(中国船舶集团有限公司第七一八研究所,河北邯郸056027)摘要:氢能是一种理想的洁净能源载体。
质子交换膜(PEM)电解水技术以其电解效率高、安全可靠、结构紧凑、对可再生能源具有快速响应性等特点,被公认为极具发展前景的制氢技术之一。
目前,制约PEM电解水技术大规模商业化应用的因素之一在于较高的制造成本,尤其是成本占比较高的贵金属,针对于PEM电解的研究多集中在贵金属催化剂上。
本文综述了析氧电催化剂研究进展,并对析氧催化剂的未来研究方向进行了展望。
关键词:PEM电解水;析氧反应;电催化剂;贵金属中图分类号:TQ153.2文献标识码:AResearch Progress of PEM Water Electrolysis Catalysts forOxygen Evolution ReactionSUN Yuetao,REN Hangxing,LI Liming,YUAN Xianming,SONG Shili,JIAO Wenqiang(The718th Research Institute of CSSC,Handan056027,China)Abstract:Hydrogen energy is an ideal clean energy carrier.Proton-exchange membranes(PEM)water electrolysis technology is recognized as one of the promising hydrogen production technologies due to its high electrolysis efficiency,safety and reliability,compact structure,and rapid response to renewable energy.At present,one of the factors restricting the large-scale commercial application of PEM water electrolysis technology is the high manufacturing cost,especially the noble metals that account for a rela⁃tively high cost.Research on PEM electrolysis is mostly concentrated on noble metal electrocatalysts. This article reviews the research progress of oxygen evolution electrocatalysts and prospects the future re⁃search directions of oxygen evolution catalysts.Keywords:PEM water electrolysis;oxygen evolution reaction;electrocatalyst;precious metal氢气是一种清洁灵活的能源载体,它可由传统的煤、石油、天然气等化石燃料制取,也可由风能、太阳能等清洁能源制取,可再生能源电解制氢被认为是生产氢气的最清洁方法,制取的氢气符合“绿氢”的概念,世界各国也都在积极推进可再生能源制氢相关技术的研究及应用。
Angew. Chem. Int. Ed. 2000, 39, 3772

1.IntroductionIn general,an ionic liquid is a liquid that consists only of ions.However,this term includes an additional special definition to distinguish it from the classical definition of a molten salt.[1]While a molten salt is generally thought to refer to a high-melting,highly viscous and very corrosive medium, ionic liquids are already liquid at low temperatures(<1008C) and have relatively low viscosity.The apparently somewhat arbitrary line drawn between molten salts and ionic liquids at a melt temperature of1008C can be justified by the abrupt improvement in the range of applications for liquid salts below this temperature.Even though some examples are known in which high-temperature salt melts have been successfully used as reaction media for synthetic applica-tions,[2]only a liquid range below1008C can enable the versatile substitution of conventional,organic solvents by ionic liquids.The development of ionic liquids goes back to1914.First research efforts dealt with the synthesis of ethylammonium nitrate.[3]This salt is liquid at room temperature but usually contains a small amount of water(200±600ppm).[4]The first ionic liquids with chloroaluminate ions were developed in1948by Hurley and Wier at the Rice Institute in Texas as bath solutions for electroplating aluminum.[5]How-ever,these systems were not studied further until the late 1970s when the groups of Osteryoung and Wilkes rediscov-ered them.For the first time,they succeeded in preparing room±temperature liquid chloroaluminate melts.[6]Research and development concentrated mainly on electrochemical applications at this time.As early as1967,a publication by Swain et al described the use of tetra-n-hexylammonium benzoate as a solvent for kinetic and electrochemical investigations.[7]Even though the liquid salt was a hemihydrate at room temperature,this research work had a pioneering significance because it already contained a quantitative determination of the ioniza-tion strength of the ionic medium.In the early1980s the groups of Seddon and Hussey began to use chloroaluminate melts as nonaqueous,polar solvents for the investigation of transition metal complexes.The investigations generally started with the electrochemical aspects of the relevant transition metal complexes;[8]spectro-scopic and complex chemistry experiments followed.[9]It is specially thanks to Seddon s work that ionic liquids became more familiar to a broad public.The first publications in which ionic liquids were described as new reaction media and catalysts for organic synthesis appeared at the end of the1980s.Acidic ionic liquids with chloroaluminate ions proved to be effective Friedel±Crafts catalysts;[10]phosphonium halide melts were used successfully in nucleophilic aromatic substitution reactions.[11]The use of ionic liquids as solvents for homogeneous transition metal catalysts was described for the first time inIonic LiquidsÐNewªSolutionsºfor Transition Metal CatalysisPeter Wa sserscheid*a nd WilhelmKeim[*]Dr.P.Wasserscheid,Prof.W.KeimInstitut für Technische Chemie undMakromolekulare Chemie der RWTH AachenWorringer Weg1,52074Aachen(Germany)Fax:( 49)241-8888177E-mail:Wasserscheidp@itc.rwth-aachen.deREVIEWSREVIEWS P.Wasserscheid and W.Keim1990by Chauvin et al.and by Wilkes et al.Chauvin s group dissolved nickel catalysts in weakly acidic chloroaluminate melts and investigated the resulting ionic catalyst solutions for the dimerization of propene.[12]Wilkes et ed also weekly acidic chloroaluminate melts and studied therein the ethylene polymerization with Ziegler±Natta catalysts.[13]The concept of ionic liquids received a substantial boost by the work of Wilkes s group when they described in1992the synthesis of systems with significantly enhanced stability against hydrolysis,for example low melting tetrafluoroborate melts.[14]In contrast to chloroaluminate ionic liquids,these systems offer high tolerance versus functional groups which opens up a much larger range of applications especially for transition metal catalysis.Ionic liquids with tetrafluoroborate ions have been successfully used,for example,in the rodium-catalyzed hydroformylation of olefins.[15]Based on Wilkes s work,it became clearly apparent that ionic liuids were by no means limited to chloroaluminate melts,quite to the contrary,a whole range of cation/anion combinations can form low-melting salts.The most recent publications are concerned with the synthesis of new ionic liquids,[16]with the systematic inves-tigation of their physical and chemical properties,[17]and with further applications as solvents and catalysts.[18]Two excellent reviews by Welton[19]and by Seddon and Holbrey[20]have been already published describing in special detail the use of chloroaluminate ionic liquids in synthetic and catalytic applications.Electrochemical[21]and complex chem-istry[22]investigations in ionic liquids have already been reviewed,too.The aim of our review is to describe the synthesis,proper-ties,and potential of ionic liquids with respect to their application as solvent in transition metal catalysis.In this context,we would like to offer especially to the chemist working in the field of homogeneous catalysis a set of criteria to identify suitable candidates out of the large number of ionic liquids(some authors speak about1018possible cation/anion combinations[20]).This section will be followed by a selection of examples which demonstrate that ionic liquids can be successful alternativeªsolutionsºfor many applications. Hereby,we will focus our attention on recently published work describing transition metal catalysis inªnon-chloroalu-minateºsystems.2.Ionic Liquid SynthesisThe initial step in the synthesis of ionic liquids is the quaternization,of an amine or phosphane for example,to form the cation.[6c,22]The most important,reported cation types are shown in Scheme1.Salts with different anionsareScheme1.Important types of cations in ionic liquids.obtained by the quaternization reaction depending on the alkylation reagent.Interestingly,melting points under1008C can be obtained for a series of cation/anion combinations in this way(Table1).REVIEWS Ionic LiquidsIn cases where it is not possible to form the desired anion directly by the quaternization reaction,a further step follows (synthesis steps IIa or IIb in Scheme 2).For example,starting from an ammonium halide [R 'R 3N] X À,two different paths to vary the anion are possible.First,the halide [R 'R 3N] X Àcan be treated with a Lewis acid MX y .This leads to an ionic liquid of the type [R 'R 3N] [MX y 1]À(synthesis step IIa,Scheme2).Scheme 2.Synthesis paths for the preparation of ionic liquids examplified for an ammonium salt.Alternatively it is possible to exchange the halide ion X Àfor the desired anion.This can be done by the addition of a metal salt M [A]À(with precipitation of M X À)over an ion exchanger or by displacement of the halide ion by a strong acid H [A]À(with the release of H X À)(synthesis step IIb,Scheme 2).In the first case,several anion species are often present in equilibrium,which depends on the ratio of the two compo-nents [R 'R 3N] X Àand MX y [Eq.(1)].With an excess of the Lewis acid MX y additional anion species can be formed from further acid ±base reactions with the already present anion.Such behavior is displayed by chloroaluminate melts,for example [Eq.(2)and (3)].[26]Theformation of different anions occurs as a function of the chloride/AlCl 3ratio.From Figure 1it can be seen that addition of aluminum trichloride to the chloride initially results in the formation of the AlCl 4Àion.With an aluminum trichloride mole fraction of exactly 0.5,this is essentially the only anion present.In systems with x (AlCl 3)>0.5multi-nuclear chloroaluminate anions are formed which are in equilibrium with each other,the AlCl 4Àion and,at very high AlCl 3contents,with dimeric aluminum trichloride.[26]Figure 1.Mole fraction x m of different anion species Xn in chloroalumi-nate melts (X1 Cl À;X4 AlCl 4À;X7 Al 2Cl 7À;X10 Al 3Cl 10À;X13 Al 4Cl 13À;X6 Al 2Cl 6).Chloroaluminates are the best known but not the only ionic liquids that can be prepared by the reaction of a halide with a Lewis acid.Further examples are shown in Table 2.When the anion is varied by anion exchange ionic liquids of the type [cation] [A]Àare formed (synthesis step IIb,Scheme 2),which contain only one anion species when the exchange reaction has proceeded to completion (Table 3).At this point it should be noted that the synthesis of highly pure,binary ionic liquids places particular demands on the Table 1.Examples of ionic liquids that can be formed by direct quater-nization.Ionic liqiud Alkylation reagent M.p.[8C]Ref.[EMIM]CF 3SO 3[a]methyl triflate À9[16a][BMIM]CF 3SO 3[b]methyl triflate 16[16a][Ph 3POc]OTs [c]OcOTs 70±71[24][Bu 3NMe]OTs MeOTs 62[25][BMIM]Cl chlorobutane 65±69[6c][a]EMIM 1-ethyl-3-methylimidazolium;CF 3SO 3 triflate anion.[b]BMIM 1-n -butyl-3-methylimidazolium.[c]Oc octyl;Ts H 3CC 6H 4-SO 2(tosyl).Table 2.Examples of ionic liquids that can be generated by the reaction of a halide with a Lewis acid.Ionic liquid [a]Established anion Ref.[cation]Cl/AlCl 3Cl À,AlCl 4À,Al 2Cl 7À,Al 3Cl 10À[27][cation]Cl/AlEtCl 2AlEtCl 3À,Al 2Et 2Cl 5À[28][cation]Cl/BCl 3Cl À,BCl 4À[29][cation]Cl/CuCl CuCl 2À,Cu 2Cl 3À,Cu 3Cl 4À[30][cation]Cl/SnCl 2SnCl 3À,Sn 2Cl 5À[31][a]cation pyridinium,imidazolium ion.Table 3.Examples of ionic liquids that can be prepared by anion exchange.Ionic liquid [a]Ref.[cation]BF 4[14,32][cation]PF 6[32,33][cation]SbF 6[30][cation]NO 3[14][cation]CH 3CO 2[14][cation]HSO 4[16f][cation]B(Et 3Hex)[34][a]cation pyridinium,imidazolium,ammonium ion.REVIEWSP .Wasserscheid and W.Keim preparative work.The purity of the system is essential for many solvent applications and for the characterization of their physical and chemical properties.Whereas organic solvents are usually purified by distillation before use,this method is not suitable to clean up ionic liquids due to their nonvolatile character.For this reason,the highest purity possible must be attained during the synthesis itself.For example,during the exchange of chloride ions for the desired anions,it must be ensured that no halide ions remain in the system.Also traces of the acid used in the synthesis can lead to unwanted chemical reactivity.High purity in the synthesis of binary ionic liquids is usually achieved by anion exchange over an ion exchanger.The described methods can,of course,also be used to prepare previously unknown combinations of cations and anions which could also result in low-melting salts.In addition there is the possibility to obtain ionic liquids with new properties by the mixture of several different salts.[35]3.Characteristic Properties of Ionic LiquidsThe physical and chemical properties of ionic liquids can be specifically varied over a wide range by the selection of suitable cations and anions.The possibility arises to optimize the ionic reaction medium for a specific application by stepwise tuning the relevant solvent properties.For this reason ionic liquids have been referred to as ªdesigner solventsºin several publications.[36]In the following section,we attempt to illustrate the relationships between the structural features of an ionic liquid and its important physical and chemical properties,on the basis of a few selected examples.3.1.Melting PointThe key criterion for the evaluation of an ionic liquid is,by definition,its melting point.Of particular significance is therefore the question of the relationship between the structure and chemical composition of an ionic liquid and its melting parison of the melting points of different chloride salts illustrates the influence of the cation clearly:High melting points are characteristic for alkali metal chlorides,whereas chlorides with suitable organic cations melt at temperatures below 1508C (Table 4).[6c,37]In the literature,the following features are discussed for cations of low-melting salts:low symmetry,[1]weak intermo-lecular interactions (such as the avoidance of hydrogen bonding),[16a,38]and a good distribution of charge in the cation.[39]Besides the cation,the anion influences the melting point,parison of the melting points of different salts with the 1-ethyl-3-methylimidazolium (EMIM)ion emphasizes that,in most cases,an increasing size of the anion with the same charge leads to a further decrease in the melting point (Table 5).For ionic liquids prepared by reaction of a halide [cati-on] X Àwith a Lewis acid MX y ,the molar ratio of the two reactants influences the melting points (Figure 2).[40]As already shown in Figure 1,a quasi-binary system with the AlCl 4Àion only exists for exact 1:1mixtures in the system [EMIM]Cl/AlCl 3.The fact that a local maximum in melting temperature is observed at exactly this composition indicates that the presence of several anions in the ionic liquid has the effect of decreasing the meltingpoint.Figure 2.Experimental phase diagram in the system [EMIM]Cl/AlCl 3(EMIM 1-ethyl-3-methylimidazolium ion).3.2.Vapor Pressure and Thermal Stability Ionic liquids have no measurable vapor pressure.This is a great advantage from a process engineering viewpoint,since separation by distillation of a reaction mixture becomes more effective as a method of product isolation.The well-known problem of azeotrope formation between the solvent and the products does not arise.The thermal stability of ionic liquids is limited by the strength of their heteroatom Àcarbon and their heteroa-tom Àhydrogen bonds,respectively.Ionic liquids synthesized by direct protonation of an amine or phosphane show,for Table 4.Melting points of various chlorides.Salt M.p.[8C]NaCl 803KCl772R R ' methyl ([MMIM]Cl)[a]125R methyl,R ' ethyl ([EMIM]Cl)87R methyl,R ' n -butyl ([BMIM]Cl)65[a]MMIM 1,3-dimethylimidazolium.Table 5.Influence of different anions on the melting point of imidazolium salts.Imidazolium salt M.p.[8C]Ref.[EMIM]Cl 87[6c][EMIM]NO 255[14][EMIM]NO 338[14][EMIM]AlCl 47[40][EMIM]BF 46[a][17d][EMIM]CF 3SO 3À9[16a][EMIM]CF 3CO 2À14[16a][a]Glass transition.REVIEWS Ionic Liquidsexample,significantly restricted thermal stability.Many melts with trialkylammonium ions already decompose at a temper-ature below808C in vacuo(depending on the boiling point of the related amine or acid).For ionic liquids obtained by alkylation of an amine or phosphane the tendency to undergo thermally induced transalkylation or dealkylation reactions (retro-quaternization reaction)is strongly related to the nature of their anion.While1508C has to be considered as maximum working temperature for most of the quaternary ammonium chloride salts,1-ethyl-3-methylimidazolium (EMIM)tetrafluoroborate,for example,has been reported to be stable to about3008C[41]and[EMIM][(CF3SO2)2N] (m.p.À38C)is stable up to even more than4008C.[16a] Consequently,some ionic liquids have,in contrast to water and most organic solvents,a liquid range of up to more than 4008C.3.3.DensityThe dependence of the density of an ionic liquid on the type of cation and anion can be illustrated clearly by the example of chloroaluminate and bromoaluminate melts.A comparison of chloroaluminate melts with different cations reveals an almost linear relationship between the density and the length of the N-alkyl chain on the imidazolium cation(Figure3).[40]Figure3.Dependence of the density1of1,3-dialkylimidazolium tetra-chloroaluminate melts on the type of both alkyl groups;measurement temperature at608C,x(AlCl3) 0.5.More generally,it can be concluded that the density of comparable ionic liquids decreases as the bulkiness of the organic cation increases.Slight structural changes in the cation allow a fine adjustment of the density.Varying the anion results in more obvious effects in several cases.With bromoaluminate melts for example,it was possible to achieve densities unusual for normal organic solvents(Figure4).[42]Density measurements of ionic liquids with triflate or trifluoroacetate ions confirmed the more general result that a certain density range is established by the choice of anion,within which a fine adjustment is possible by careful choice of the cation.[16a]3.4.ViscosityThe viscosity of ionic liquids is essentially determined by their tendency to form hydrogen bonding and by the strength of their van der Waals interactions.[16a]Figure4.Dependence of the density1of two1-ethyl-3-methylimidazo-lium tetrahaloaluminate melts on the mole fraction of aluminum trihalide at608C.The effect of hydrogen bonding becomes clear when,for example,the viscosities of chloroaluminate melts of different compositions are compared(Figure5).[40]The increase in viscosity of more than a factor of ten in ionic liquidswithFigure5.Dependence of the dynamic viscosity h[cP]of two1,3-dialkylimidazolium tetrachloroaluminate melts on the mole fraction of aluminum trichloride at258C.x(AlCl3)<0.5is a result of the formation of hydrogen bonds between the hydrogen atoms of the imidazolium cation and the basic chloride ion.This statement is supported by IR[43] and X-ray spectroscopy,[44]ROESY-NMR,and theoretical calculations.[45]In acidic mixtures,however,the anions AlCl4Àand Al2Cl7Àare present,in which the negative charge is much better distributed.This leads to the formation of weaker hydrogen bonds and a much lower viscosity. Comparison of the viscosity of different,hydrophobic ionic liquids with1-n-butyl-3-methylimidazolium(BMIM)ions emphasizes,in addition,the interplay between van der Waals interactions and hydrogen bonding(Table6).[16a]The transi-tion from the triflate ion to the n-C4F9SO3Àion,and from the trifluoroacetate ion to the n-C3F7COOÀion reveals anTable6.Dynamic viscosities h of various1-n-butyl-3-methylimidazolium (BMIM)salts at208C.Anion[A]Àh[cP]CF3SO3À90n-C4F9SO3À373CF3COOÀ73n-C3F7COOÀ182(CF3SO2)2NÀ52REVIEWS P.Wasserscheid and W.Keimobvious increase in viscosity.It is apparent that the stronger van der Waals interactions in the case of the n-C4F9SO3Àand n-C3F7COOÀions result in a higher viscosity of the ionic parison of the viscosities of[BMIM]CF3SO3with [BMIM](CF3SO2)2N,reveals a lower viscosity despite stron-ger van der Waals interactions for ionic liquids with the (CF3SO2)2NÀion.In this case,the almost complete suppres-sion of hydrogen bonding overcompensates for the expected increase in viscosity.[16a]The structure of the cation also influences the viscosity of the ionic liquid.The lowest viscosities are usually obtained for melts with the1-ethyl-3-methylimidazolium(EMIM)ion,in which a side chain with sufficient mobility is combined with a low molar mass.Longer or fluorinated alkyl chains result in higher viscosities because of stronger van der Waals inter-actions.[16a]The viscosity of ionic liquids can be lowered,drastically in some cases,by only slight increases in temperature[40,46]or by the addition of small amounts of organic cosolvents.[47]3.5.Solvation Strength and Solubility CharacteristicsThe tuning of solubility properties of an ionic liquid by the careful choice of cation and anion deserves particular attention.The influence of the cation,for example,is shown by investigations of the solubility of1-octene in different tosylate melts(Figure6).[25]It can be seen that with increasing nonpolar character of the cation,the solubility of1-octene in the melt increases markedly.In methyl-tri-n-octylammo-nium tosylate a single-phase reaction mixture is obtained.This example shows that stepwise variation of the solubility properties can be achieved by variation of the alkyl group on the cation.The influence of the anion on the solubility characteristics of ionic liquids can be demonstrated in an impressive fashion by the example of the water solubility of different melts containing the BMIM ion.While[BMIM]Br,[BMIM]-CF3COO,and[BMIM]CF3SO3are highly water-soluble,ionic liquids with the same cation but with a PF6Àor(CF3SO2)2NÀion form biphasic mixtures with water.The water content of the ionic liquid[BMIM](CF3SO2)2N at208C is only1.4weight percent.[16a]Several ionic liquids showing a miscibility gap with water have been considered as interesting candidates for separation processes by liquid±liquid extraction.Rogers et al.inves-tigated,for example,the solubility of different acids and bases in water/[BMIM]PF6at different pH values of the aqueous phase.[48]Interestingly,their results reveal a higher solubility of neutral substrates in the ionic liquid,while ionic species dissolve preferentially in the aqueous layer.The authors conclude that the solubility properties of[BMIM]PF6versus water show high similarity to organic solvents.The substitu-tion of volatile organic solvents by ionic liquids in extractive separation processes may therefore be an interesting option. Many ionic liquids are completely miscible with organic solvents if their dielectric constants exceed a characteristic limit.This limit appears to be specific for each cation/anion combination(Table7).[16a]The solubility of supercritical CO2in[BMIM]PF6that has recently been investigated by Brennecke s group is remark-able,too.[49]In the biphasic system scCO2/[BMIM]PF6 60mol%of CO2dissolve in the ionic liquid at80bar CO2 pressure.Hereby,the volume of the ionic liquidincreases only by10±20%,however.As a firstapplication of this interesting biphasic system,theauthors investigated the extraction of naphthalenefrom the ionic liquid.They succeeded in recoveringthe naphthalene quantitatively without any detect-able contamination of the extract by the ionic liquid.Without doubt,the key to the successful use ofionic liquids lies in the skillful exploitation of theirexceptional solubility characteristics.Further system-atic investigations are necessary,however,to take fulladvantage of this huge potential.One very promising possibility is,for example,theinvestigation of the polarity of ionic liquids.Thepolarity of a solvent is usually determined in a purelyempirical fashion.A well-understood,easily measur-able and strongly solvent-dependent process is deter-Figure6.Solubility of1-octene in four different tri-n-alkylmethylammonium tosylatemelts at808C.n(C) number of C atoms of the alkyl residue.Table7.Miscibility of various ionic liquids with the1-ethyl-3-methylimidazolium(EMIM)ion in organic solvents with the dielectric constant e.[a] Solvent e[EMIM]CF3SO3[EMIM]CF3COO[EMIM]n-C3F7COO[BMIM]CF3COO[b][BMIM]n-C3F7COO CH2Cl28.93m m m m mTHF7.58m m m m methyl acetate 6.02m pm pm m mtoluene 2.38im im im im im1,4-dioxane 2.01im im im im im[a]m:miscible;pm:partially miscible;im:immiscible.REVIEWS Ionic Liquids mined in a large number of different solvents,for example the spectral absorption of a solvatochromic dye.Empirical solvent polarity parameters are derived from the measured absorption maxima,which reflect the solvating ability of a solvent much more comprehensively than the individual physical constants.[50]Amongst the many empiri-cal polarity scales,the E (T)(30)scale introduced by Dimroth et al.in 1963[51]and further devel-oped by Reichhardt et al.in 1971has proven successful,and is based on the solvatochromism of a pyridinium-N -phenolate betaine dye.[52]This method has also been used successfully to determine the polarity of a small number of ionic liquids.The results obtained confirm the considerable width of variation of solvent polarity of ionic liquids.While,for example,tetra-n -hexylammonium benzoate with an E (T)(30)value of 0.41lies within the polarity range of DMF,[53]an E (T)(30)value of 0.95was determined for ethyl-ammonium nitrate corresponding to a polarity between CF 3CH 2OH and water.[4,54][BMIM]PF 6was investigated by using the same method and its E (T)(30)value was found to correspond to a polarity in the range of methanol.[55]However,recent work by Armstrong et al.gave rise to the question whether the chemical nature of the solvatochromic dye influences the result of the polarity determination of an ionic liquid.These authors coated GC columns with several ionic liquids and compared the retention times of a large number of substances.[56]The results of their study indicate different polarity of ionic liquids depending on the nature of the tested compounds.While [BMIM]PF 6,for example,acts like an unpolar stationary phase versus unpolar molecules (like n -octane),very long retention times are observed with proton-donor compounds.The authors attribute a dual polarity behavior to the ionic liquids under investigation.Unfortunately the number of systematic investigations concerning the polarity of ionic liquids is still very limited.More research in this field should be encouraged in order to establish efficient criteria to determine the right ionic liquid candidate for a given solvent application.3.6.Acidity and Coordination AbilityThe acidity and coordination properties of an ionic liquid are essentially determined by the nature of its anion.Many intermediate levels between ªstrongly basic/strongly coordi-natingºand ªstrongly acidic/practically noncoordinatingºcan be realized by careful choice of the anion (Table 8).[30]In this context,those ionic liquids have to be mentioned which form a neutral anion (e.g.AlCl 4À)or an acidic anion (e.g.Al 2Cl 7À)from a basic anion (e.g.Cl À)by addition of a Lewis acid (e.g.AlCl 3).In Scheme 3this behavior is illustrated by the example of a EMIM chloroaluminate melt.Chloroaluminate melts are designated as basic when the molar ratio of AlCl 3is smaller than 0.5.A neutral melt is referred to at an AlCl 3ratio of exactly 0.5,where essentially only the anion AlCl 4Àis present.[22a]Finally,an acidic chloroaluminate melt is one in which the AlCl 3ratio is larger than 0.5.In such acidic melts,the anions Al 2Cl 7Àand Al 3Cl 10Àexist,which act as very strong Lewis acids.[27]Two further phenomena in the field of acid/base chemistry of ionic liquids deserve to be mentioned.These are the so-called ªlatent acidityºand ªsuperacidityºof protons in ionic liquids.The latent acidity of ionic liquids arises when weak bases are added to buffered neutral chloroaluminate melts.Such melts are formed when,for example,excess alkali metal chloride (MCl)is added to an acidic chloroaluminate melt.[57]The alkali metal chloride MCl reacts according to Equa-tion (4)with the acidic chloroaluminate dimers until themelt becomes neutral.A buffered melt is one in which the neutrality of the melt is maintained by reaction of excess alkali metal chloride when acid AlCl 3is added.The latent acidity of this neutral system becomes noticeable when a weak base (B)such as N ,N -dimethylaniline,pyrrole,or acetylferro-cene is added.[58]An adduct is formed between the added base and AlCl 3with precipitation of the alkali chloride MCl [Eq.(5)].This reaction is not observed in the absence of excess alkali metal cations.The latent acidity of different ionic liquids has already been quantitatively measured.[59]In our group,ionic liquids with latent acidity have been successfully used as solvents in the Ni-catalyzed oligomerization of 1-butene (see Section 4.8).[60]The superacidity of protons in several ionic liquids is also worth mentioning.It has been observed when strong mineral acids were dissolved in acidic chloroaluminate ionic liquids.[61]Smith and co-workers investigated the acidity of such protons in ionic liquids by the protonation of aryl compounds with a solution of HCl gas in acidic [EMIM]Cl/AlCl 3melts.The acidity of the protons in the melt were measured quantita-Table 8.Coordinative characteristics of various anions.Acidity/coordination basic/strongly coordinating neutral/weakly coordinating acidic/non-coordinatingCl ÀAlCl 4ÀAl 2Cl 7ÀAc ÀCuCl 2ÀAl 3Cl 10ÀNO 3ÀSbF 6ÀSO 42ÀBF 4ÀCu 2Cl 3ÀPF 6ÀCu 3Cl 4ÀScheme 3.Control of the acidity of ionic liquids by the ratio of halide to Lewis acid examplified for 1-ethyl-3-methylimidazolium (EMIM)chloroaluminate melt.。
红外光谱和X-射线衍射表征水铁矿吸附砷酸的性质
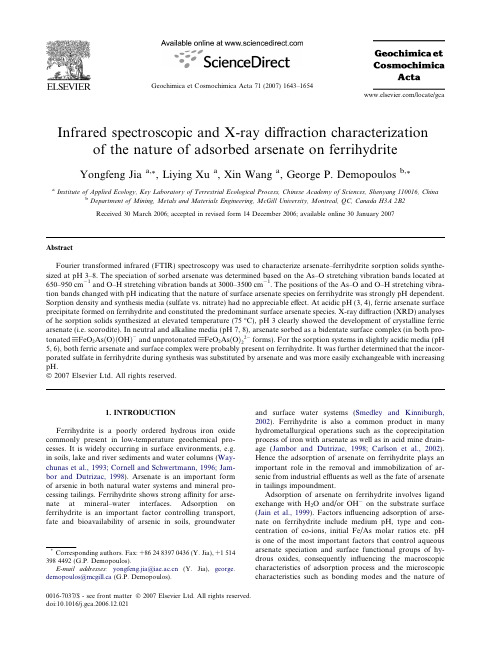
Infrared spectroscopic and X-ray diffraction characterizationof the nature of adsorbed arsenate on ferrihydriteYongfeng Jiaa,*,Liying Xu a ,Xin Wang a ,George P.Demopoulosb,*aInstitute of Applied Ecology,Key Laboratory of Terrestrial Ecological Process,Chinese Academy of Sciences,Shenyang 110016,ChinabDepartment of Mining,Metals and Materials Engineering,McGill University,Montreal,QC,Canada H3A 2B2Received 30March 2006;accepted in revised form 14December 2006;available online 30January 2007AbstractFourier transformed infrared (FTIR)spectroscopy was used to characterize arsenate–ferrihydrite sorption solids synthe-sized at pH 3–8.The speciation of sorbed arsenate was determined based on the As–O stretching vibration bands located at 650–950cm À1and O–H stretching vibration bands at 3000–3500cm À1.The positions of the As–O and O–H stretching vibra-tion bands changed with pH indicating that the nature of surface arsenate species on ferrihydrite was strongly pH dependent.Sorption density and synthesis media (sulfate vs.nitrate)had no appreciable effect.At acidic pH (3,4),ferric arsenate surface precipitate formed on ferrihydrite and constituted the predominant surface arsenate species.X-ray diffraction (XRD)analyses of he sorption solids synthesized at elevated temperature (75°C),pH 3clearly showed the development of crystalline ferric arsenate (i.e.scorodite).In neutral and alkaline media (pH 7,8),arsenate sorbed as a bidentate surface complex (in both pro-tonated B FeO 2As ðO ÞðOH ÞÀand unprotonated B FeO 2As ðO Þ22Àforms).For the sorption systems in slightly acidic media (pH 5,6),both ferric arsenate and surface complex were probably present on ferrihydrite.It was further determined that the incor-porated sulfate in ferrihydrite during synthesis was substituted by arsenate and was more easily exchangeable with increasing pH.Ó2007Elsevier Ltd.All rights reserved.1.INTRODUCTIONFerrihydrite is a poorly ordered hydrous iron oxide commonly present in low-temperature geochemical pro-cesses.It is widely occurring in surface environments,e.g.in soils,lake and river sediments and water columns (Way-chunas et al.,1993;Cornell and Schwertmann,1996;Jam-bor and Dutrizac,1998).Arsenate is an important form of arsenic in both natural water systems and mineral pro-cessing tailings.Ferrihydrite shows strong affinity for arse-nate at mineral–water interfaces.Adsorption on ferrihydrite is an important factor controlling transport,fate and bioavailability of arsenic in soils,groundwaterand surface water systems (Smedley and Kinniburgh,2002).Ferrihydrite is also a common product in many hydrometallurgical operations such as the coprecipitation process of iron with arsenate as well as in acid mine drain-age (Jambor and Dutrizac,1998;Carlson et al.,2002).Hence the adsorption of arsenate on ferrihydrite plays an important role in the removal and immobilization of ar-senic from industrial effluents as well as the fate of arsenate in tailings impoundment.Adsorption of arsenate on ferrihydrite involves ligand exchange with H 2O and/or OH Àon the substrate surface (Jain et al.,1999).Factors influencing adsorption of arse-nate on ferrihydrite include medium pH,type and con-centration of co-ions,initial Fe/As molar ratios etc.pH is one of the most important factors that control aqueous arsenate speciation and surface functional groups of hy-drous oxides,consequently influencing the macroscopic characteristics of adsorption process and the microscopic characteristics such as bonding modes and the nature of0016-7037/$-see front matter Ó2007Elsevier Ltd.All rights reserved.doi:10.1016/j.gca.2006.12.021*Corresponding authors.Fax:+862483970436(Y.Jia),+15143984492(G.P.Demopoulos).E-mail addresses:yongfeng.jia@ (Y.Jia),george.demopoulos@mcgill.ca (G.P.Demopoulos)./locate/gcaGeochimica et Cosmochimica Acta 71(2007)1643–1654adsorbed arsenate on the surface of ferrihydrite(Mas-scheleyn et al.,1991;Hsia et al.,1992;Fuller et al., 1993;Bowell,1994;Wilkie and Hering,1996;Raven et al.,1998;Jain et al.,1999;Meng et al.,2000;Grafe et al.,2002;Dixit and Hering,2003).The degree of pro-tonation of arsenate anion in aqueous solution is a func-tion of pH with p K a1=2.3,p K a2=6.8and p K a3=11.6 (Goldberg and Johnston,2001),resulting in arsenate spe-cies varying from H3AsO4,H2AsO4À,HAsO42À,toAsO43Àwhen pH increases from acidic region to alkalineregion(Myneni et al.,1998;Raven et al.,1998;Goldberg and Johnston,2001).On the other hand,the presence and the density of surface groups of ferrihydrite,i.e. H2O,OHÀ,are also strongly pH dependent.The point of zero charge(PZC)is approximately8.5(Jain et al., 1999;Goldberg and Johnston,2001).Hence,the modes of complexation of arsenate anions with ferrihydrite by replacing surface hydroxyl groups and/or waters are lar-gely controlled by the pH of reaction medium.Ligand exchange in the mode of bidentate binuclear in-ner-sphere complexation is the widely accepted mechanism of the adsorption of arsenate on iron oxides.It has been proposed based on infrared(Harrison and Berkheiser, 1982)and extended X-ray absorptionfine structure(EX-AFS)(Waychunas et al.,1993)analyses and confirmed to be the dominant interaction mode of arsenate–ferrihydrite and arsenate–goethite systems(Lumsdon et al.,1984;Man-ceau,1995;Sun and Doner,1996;Waychunas et al.,1996; Fendorf et al.,1997;Foster et al.,1998;Myneni et al.,1998; O’Reilly et al.,2001;Roddick-Lanzilotta et al.,2002;Sher-man and Randall,2003;Arai et al.,2004;Cance`s et al., 2005;Waychunas et al.,2005).However,most of the stud-ies were conducted at neutral to alkaline pH.The applica-bility of the conclusions to the acidic arsenate–ferrihydrite adsorption system may be questionable.Moreover,most of the studies have dealt mainly with characterizing the bonding mechanism between arsenate anions and surface iron polyhedra without identifying arsenate species on the surface of iron oxide.Based on macroscopic measurements of the adsorption process,arsenate was adsorbedas B FeO2AsðOHÞ2;B FeO2AsðOÞðOHÞÀ;B FeO2AsðOÞ22Àon the surface of ferrihydrite at mildly acidic and alkaline pH(Jain et al.,1999).In a recent work on direct character-ization of arsenate coordination on mineral(portlandite, gibbsite,ettringite,Fe-oxyhydroxides)surfaces using FTIR, both protonated and unprotonated arsenate species(i.e.HAsO42Àand AsO43Àwere present on the goethite surfaceat alkaline pH(Myneni et al.,1998).When arsenate was ad-sorbed on schwertmannite and ferrihydrite at acidic pH(i.e. pH3),surface precipitates were proposed to form and were termed as ferric hydroxyarsenate(FeOHAs)(Carlson et al., 2002).Evidence for surface precipitation of phosphate on goethite has been observed(Ler and Stanforth,2003).Sim-ilarly,surface precipitation of ferric arsenate on ferrihydrite is likely to occur in addition to bidentate binuclear com-plexation according to the XRD and Raman spectroscopic evidence we reported recently(Jia et al.,2006).The objective of this paper was to provide further evi-dence of surface precipitation of ferric arsenate on ferrihy-drite.This was done via characterization of the interactions between arsenate and ferrihydrite in terms of bonding modes and surface arsenate species as a function of pH and coverage density by Fourier transformed infrared spec-troscopy(FTIR)and evolution of crystallinity at elevated temperature(75°C)by X-ray diffraction(XRD)analysis. The effect of ferrihydrite synthesis media(NO3Àvs.SO42À)was also evaluated since it significantly influenced arsenate adsorption capacity(Jia and Demopoulos,2005). Poorly crystalline ferric arsenate was used as reference material in the study.Moreover,relatively high arsenic con-centration solutions were used in this study since this is the case in important hydrometallurgical operations where arsenic removal is practiced.2.MATERIALS AND METHODS2.1.Synthesis of poorly crystalline ferric arsenatePoorly crystalline ferric arsenate was synthesized at 21°C by adjusting a0.02M As(V)/0.02M Fe(III)solution (as sodium arsenate and ferric sulfate)from initial pH1.3to 1.8with NaOH solution and maintained at that pH for1h (Jia et al.,2006).The resultant solid was separated byfiltra-tion,washed with de-ionized water(pH2)and vacuum-dried at60°C.The chemical formula(Fe1.02AsO4Æ2.4H2O) was determined by digestion with hydrochloric acid fol-lowed by ICP-AES analysis.2.2.Synthesis of arsenate–ferrihydrite sorption samplesTwo-line ferrihydrite samples were synthesized at21°C using a slightly modified procedure from that reported in the literature(Schwertmann and Cornell,1991).Both ni-trate(Fe(NO3)3Æ9H2O)and sulfate(Fe2(SO4)3Æ5H2O)salts were used as the sources of ferric iron[Fe(III)].Briefly, the Fe(III)solution was prepared by dissolving ferric ni-trate or ferric sulfate in de-ionized water.The pH of the solution was raised to$7.5in about5min using1M NaOH solution and maintained at that pH for1h with the slurry mechanically agitated vigorously.The ferrihy-drite samples synthesized from sulfate and nitrate media were termed as‘‘sulfate–ferrihydrite’’and‘‘nitrate–ferrihy-drite’’,respectively,for simplicity.The prepared ferrihydrite slurry was adjusted to differ-ent pH between3and8with NaOH and HNO3and al-lowed to equilibrate for1h.Arsenate solution was introduced into the ferrihydrite slurry from a burette over a10-min period with the slurry mechanically stirred moder-ately.The pH was controlled by addition of NaOH and/or HNO3solution and allowed to equilibrate at21°C for 2weeks.The volume of adsorption slurry was500mL for all experiments and the concentration of Fe(III)in the slur-ry system was4g/L.At each pH,three initial Fe/As molar ratios(i.e.Fe/As=2,4and8)were applied.Arsenate–fer-rihydrite sorption samples were also synthesized at75°C, Fe/As molar ratio of2and4.The pH of the slurry was con-trolled constant at pH3throughout the sorption reaction. Samples were taken at1day,3day,1week,2weeks and 2months of reaction time.The synthesized arsenate–fer-rihydrite sorption products werefiltered,DI water-rinsed1644Y.Jia et al./Geochimica et Cosmochimica Acta71(2007)1643–1654and vacuum-dried at60°C.The equilibrium concentration of arsenic was determined by ICP-AES analysis.2.3.FTIR analysisThe infrared spectra of the samples were obtained on a Bio-Rad FTS60Fourier Transformed Infrared Spectrome-ter with a MCT liquid nitrogen cooled detector.The KBr/ sample discs were prepared by mixing0.5%offinely ground samples in KBr.The sample chamber was purged by N2gas for10min before scans were started.The measurement res-olution was set at4cmÀ1and the spectra were collected in the range of400–4000cmÀ1with200co-added scans.2.4.X-ray diffraction(XRD)analysisThe powder XRD patterns were collected on a Rigaku D/Max2500PC X-ray diffractometer with graphite mono-chromated CuK a1radiation.The powder samples were scanned from10to90°2h with increments of0.02°2h.3.RESULTS AND DISCUSSION3.1.Effect of pH on the nature of adsorbed arsenateAqueous arsenate species have no direct bearing on the surface arsenate species adsorbed on mineral surfaces (Myneni et al.,1998).However,the effect of complexation of arsenate ions on oxide surfaces is similar to that of pro-tonation of aqueous arsenate species.A brief discussion on infrared absorption of aqueous arsenate can assist with understanding the infrared characteristics of surface species (Myneni et al.,1998;Goldberg and Johnston,2001;Rod-dick-Lanzilotta et al.,2002).The free arsenate anion,AsO43À,is present in highly alkaline(p K a3=11.6)aqueoussolution and belongs to T d symmetry.Only m3and m4funda-mental bands are infrared active in this form.The infrared spectrum of an AsO43Àdominated solution exhibits a major band at792cmÀ1(Roddick-Lanzilotta et al.,2002).Uponprotonation or complexation with metal cations,the sym-metry decreases and splitting of the m3band occurs(Harri-son and Berkheiser,1982;Myneni et al.,1998).AqueousHAsO42Àspecies belong to the C3v symmetry.Its infrared spectrum shows two broad bands at859and689cmÀ1, the latter was assigned to stretching vibration of As–OH (Myneni et al.,1998).Curvefitting of the former band gave two bands at865and846cmÀ1,which were assigned to asymmetric and symmetric stretching vibration of uncom-plexed As–O,respectively(Myneni et al.,1998).Poorly crystalline ferric arsenate was used as reference material in this study to identify the possible occurrence of a surface precipitate of arsenate on ferrihydrite.This compound is not well defined and often termed loosely as amorphous ferric arsenate(Krause and Ettel,1989)or amorphous scorodite(Langmuir et al.,1999),because it possesses similar bonding structures to crystalline ferric arsenate,i.e.scorodite(FeAsO4Æ2H2O).It is an unstable arsenate phase with increasing pH and tends to convert to ferrihydrite(Krause and Ettel,1989).The poorly crystalline ferric arsenate synthesized in this work was determined to have the formula Fe1.02AsO4Æ2.4H2O.Fig.1shows the effect of pH on the infrared spectra of the sorption samples of arsenate on sulfate–ferrihydrite(initial molar ratio of Fe/As=2).Both detailed display of the As–O stretching vibration region(500–1000cmÀ1)and the whole range of the scanning(400–4000cmÀ1)are shown in thefigure.Poorly crystalline ferric arsenate shows a strong well-resolved band at838cmÀ1.Within the crystalline ferric arsenate(i.e.scorodite)structure,AsO4tetrahedra and FeO4(OH2)2octahedra connect alternately at vertices(Kita-hama et al.,1975).The arsenate is coordinated with four iron octahedra with an average As–O bond length of1.68A˚.The band at838cmÀ1was attributed to the stretching vibration of As–O coordinating to iron atom,i.e.As–O–Fe.The weakThe nature of adsorbed arsenate on ferrihydrite1645shoulder at$750cmÀ1was probably caused by the hydrogen bonding between H2O and AsO4since the H-bonding re-sulted in increased bond length and a red shift of the wave number(Myneni et al.,1998).The1625cmÀ1band was due to water O–H bending mode whereas the stretching vibration bands of O–H were located at$3194and$3373cmÀ1.The bands between950and1250cmÀ1were assigned to struc-tural SO42Àions,which were incorporated into the poorlycrystalline ferric arsenate by substitution of AsO43Àions dur-ing synthesis from sulfate medium.Sulfate ions were incorpo-rated into crystalline scorodite synthesized from sulfate solution(Singhania et al.,2005).The infrared spectra of pH3and4sorption samples also exhibited a strong,well-resolved band in the As–O stretching vibration region at similar position($833cmÀ1)to that of poorly crystalline ferric arsenate,indicating similarities of the arsenate bonding structures between sorption samples and poorly crystalline ferric arsenate.This suggested the for-mation of a ferric arsenate surface precipitate in the arsenate–ferrihydrite sorption samples synthesized in acidic media. However,the weak shoulder at$750cmÀ1on the FTIR spec-trum of the poorly crystalline ferric arsenate was missing for the sorption solids for some unknown reasons.The band at$833cmÀ1for the pH3and4sorption samples was assigned to As–O stretching vibration of the As–O–Fe coordination of ferric arsenate precipitate on fer-rihydrite.The formation of ferric arsenate phase in pH3 and4sorption samples was also supported by the O–H stretching vibration band at$3190cmÀ1.All samples showed a strong broad O–H band at$3370,but only theacidic sorption samples displayed the$3190cmÀ1O–H stretching vibration band like the case of poorly crystalline ferric arsenate.This characteristic O–H band of poorly crystalline ferric arsenate at$3190cmÀ1was fading out with increasing pH,indicating the disappearance of ferric arsenate surface precipitate at neutral and alkaline pH.As pH increased from3to8,the As–O stretching vibra-tion band shifted gradually from$833cmÀ1down to $806cmÀ1.At the same time,a new band emerged at high-er frequency(870–880cmÀ1)and its intensity was more pronounced with increasing pH.At pH8,we could clearly see the splitting of the single band into two bands.Peak deconvolution and curvefitting of the band produced two peaks at$806–810and$878cmÀ1(Fig.2).It is well estab-lished that at mildly alkaline pH,arsenate is adsorbed on ferrihydrite via bidentate binuclear complexation with surface iron polyhedra(Harrison and Berkheiser,1982; Waychunas et al.,1993).The band at$878cmÀ1of the pH6–8sorption samples was assigned to uncomplexed/ unprotonated As–O,whereas the$806–808cmÀ1arose from the two As–O–Fe complexed to ferrihydrite surface. Two infrared bands at824/861and817/854cmÀ1were ob-served for the arsenate adsorbed on amorphous iron oxide at pH5and9,respectively(Goldberg and Johnston,2001). The lower frequency bands at817and824cmÀ1were as-signed to the stretching vibration of As–O–Fe and the high-er frequency bands at854and861cmÀ1were attributed to ‘‘non-surface-complexed’’As–O bonds of the adsorbed arsenate species(Goldberg and Johnston,2001).It is inter-esting to note that the lower frequency band increased from 817and824cmÀ1as pH increased from5to9.This obser-vation and the band assignments are in good agreement with present work.Roddick-Lanzilotta et al.(2002)also reported that the As–O stretching vibration band of the ad-sorbed arsenate on ferrihydrite shifted from$825to $800cmÀ1as pH increased from2.6to8.In the case of bidentate binuclear complexation,two of the four As–O bonding structures are complexed to iron atoms(i.e.As–O–Fe)and the remaining two are present either both as unprotonated As–O or one as unprotonated As–O and the other one as protonated As–O–H.In com-parison,arsenate ions are coordinated to four iron atoms in ferric arsenate.According to Myneni et al.(1998),the force constant of the As–OM bond increases with coordina-tion number and decreases compared to uncomplexed As–O.Hence,for the bidentate adsorbed arsenate ion,the force constant of the two coordinated As–O–Fe is lower than that of the As–O–Fe in ferric arsenate,whereas the uncomplexed/unprotonated As–O bond has larger force constant compared to ferric arsenate.Consequently,the stretching vibration frequency of the uncomplexed/unprot-onated As–O is located at higher position while the fre-quency of the complexed As–O–Fe band is located at lower position.The increase and decrease of the As–O force constant for the bidentate binuclear complexed arsenate compared to ferric arsenate is supported by the As–O bond length(two at1.62,1.67A˚and the other two at1.71A˚, compared to1.68A˚of ferric arsenate)(Sherman and Ran-dall,2003).The shorter bond distance results in a stronger force constant and consequently higher infrared frequency.1646Y.Jia et al./Geochimica et Cosmochimica Acta71(2007)1643–1654A very weak band was observed at$700cmÀ1on the infrared spectrum of pH8arsenate–ferrihydrite sorption sample(see Fig.1).This band was reasonably assigned to protonated As–O–H bond of the adsorbed arsenate species, which was located at similar position to that of aqueous protonated arsenate species(Myneni et al.,1998).Complex-ation with metals cannot give such a low As–O stretching vibration frequency.The presence of protonated arsenate species was also proposed for the adsorption of arsenate on freshly prepared hydrous iron oxide and goethite(Myn-eni et al.,1998).It was noted that the weak As–O–H band at$700cmÀ1was absent at acidic pH indicating the ab-sence of protonated adsorbed arsenate species on ferrihy-drite at acidic pH.In a previous study using a dispersion infrared instrument(Harrison and Berkheiser,1982),three bands at875,805and700cmÀ1were observed for the ad-sorbed arsenate on hydrous ferric oxide(HFO)at pH6.5. They are very similar to the infrared bands of the pH8 sorption samples of this work(878,806and700cmÀ1).pH3and8are the extreme cases for the adsorption of arsenate on ferrihydrite in this work.At pH3,a sur-face precipitate developed and the adsorbed arsenate spe-cies were present mainly as poorly crystalline ferric arsenate.The possibility of surface precipitation of arse-nate on ferrihydrite was also suggested previously by Stanforth(1999)and Carlson et al.(2002).According to the latter research,a poorly crystalline ferric hydroxy-arsenate(FeOHAs)surface precipitate was found to form during adsorption of arsenate on schwertmannite and fer-rihydrite at pH3(Carlson et al.,2002).Similarly,surface precipitation of phosphate on goethite has been proposed in recent studies(Zhao and Stanforth,2001;Ler and Stanforth,2003).It was suggested that the adsorption reaction consisted of two phases:thefirst phase of rapid surface complexation followed by the second phase of slow buildup of a surface precipitate(Zhao and Stan-forth,2001).At the other extreme(i.e.pH8)arsenate was adsorbed via bidentate binuclear complexation with surface iron atoms in the form of unprotonated and probably protonated arsenate species as well(i.e.B FeO2AsðOÞ22Àand B FeO2AsðOÞðOHÞÀ, where B Fe represents the surface of ferrihydrite).This is in good agreement with the model proposed previously by Myneni et al.(1998),Jain et al.(1999)and Goldberg and Johnston(2001).Myneni et al.(1998)suggested that both protonated and unprotonated arsenate species were present as surface arsenate species adsorbed on ferrihydrite at alkaline pH.The surface arsenate specieswere proposed to be XH2AsO4,XHAsO4À,XAsO42À(X was Al or Fe)(Jain et al.,1999;Goldberg and Johnston, 2001).As pH increased from3to8,surface arsenate species shifted from poorly crystalline ferric arsenate precipitates to bidentate surface complexes.This is reasonable since poorly crystalline ferric arsenate is stable only at acidic pH and decomposes with increasing pH(Krause and Ettel, 1989).For the sorption systems whose media pHs lay be-tween3and8,both types of arsenate species were probably present on the surface of ferrihydrite,with the feature of poorly crystalline ferric arsenate being more pronounced at lower pH and the feature of bidentate complexes being more pronounced at higher pH.Similar to the Fe/As=2systems,the single As–O stretching vibration band shifted down gradually and split into two bands as pH increased from3to8for the Fe/As= 4sorption samples(Fig.3).The single band at acidic pH was assigned to As–O–Fe of ferric arsenate surface pre-cipitate.The presence of$3190cmÀ1O–H stretching vibra-tion band also indicated the development of ferric arsenate in the sorption solids at acidic pH.This band was absent onThe nature of adsorbed arsenate on ferrihydrite1647the infrared spectra of sorption samples at alkaline pH.The two bands(808and878cmÀ1)at alkaline pH are attributed to As–O–Fe bidentate–binuclear coordinating to ferrihy-drite and the uncomplexed/unprotonated As–O bond, respectively.The presence of protonated As–O–H bond could not be ruled out,since there appeared to be a weak feature at$700cmÀ1.It was interesting to note that all sorption samples from pH3to8had very similar sorption density(i.e.As/Fe$0.25,see Table1),but the surface arse-nate species varied from poorly crystalline ferric arsenate to bidentate–binuclear surface complexes as pH increased. This was indicative that the nature of surface arsenate spe-cies strongly depended on the pH of the sorption media.The spectra of pH3and4sorption samples showed strong sulfate bands between950and1250cmÀ1.Sulfate ions were apparently adsorbed onto the ferrihydrite during synthesis from sulfate medium and displaced by arsenate during adsorption as discussed elsewhere(Jia and Demopo-ulos,2005).At higher pH(i.e.5–8),the sulfate ions were substituted by arsenate ions as indicated by the absence of sulfate bands on the infrared spectra.For the adsorption systems of Fe/As=2,no sulfate band was observed for all pH samples(see Fig.1).Ferrihydrite synthesized from sulfate media was found to adsorb significantly more arsenate than that from nitrate media(Jia and Demopoulos,2005),which could not be ex-plained by the difference in BET surface areas.Therefore,it was of interest to compare the surface arsenate species of the two types of arsenate–ferrihydrite sorption samples. Fig.4shows the effect of pH on the infrared spectra of arsenate adsorbed on nitrate–ferrihydrite.Apparently the spectra are similar to those of arsenate adsorbed on sul-fate–ferrihydrite(Fig.1).Both are dominated by the strong As–O stretching vibration bands at700–950cmÀ1and strong O–H stretching vibration bands at3000–3500cmÀ1.In the As–O stretching vibration region(700–950cmÀ1),the infrared spectra of acidic sorption samples (pH3,4)exhibited a strong,well-resolved single band at similar position to that of poorly crystalline ferric arse-nate.This was indicative that a ferric arsenate surface precipitate formed when arsenate adsorbed on nitrate–fer-rihydrite at acidic pH,similar to As(V)adsorption onTable1Arsenic equilibrium concentration(mg/L)and sorption density(mol-As/mol-Fe)for the adsorption of arsenate on ferrihydrite synthesized from sulfate and nitrate medium at pH3–8and initial Fe/As molar ratio of2,4and8pH Fe/As=2Fe/As=4Fe/As=8Sulfate Nitrate Sulfate Nitrate Sulfate3198(0.49)318(0.46) 1.0(0.25) 1.9(0.25)<0.02(0.125) 4321(0.46)471(0.43) 1.9(0.25) 3.4(0.25)0.2(0.125) 5440(0.44)615(0.41) 3.6(0.25)14.8(0.25)0.2(0.125) 6498(0.43)788(0.38) 5.0(0.25)29.6(0.25)0.2(0.125) 7702(0.40)1070(0.32)15.8(0.25)100(0.24)0.6(0.125) 81178(0.30)1439(0.25)141(0.24)178(0.23) 2.1(0.125)The numbers in bracket are sorption densities,i.e.As/Fe molar ratio of the solids.1648Y.Jia et al./Geochimica et Cosmochimica Acta71(2007)1643–1654sulfate–ferrihydrite.As pH increased,the single As–O stretching vibration band split into two strong bands at $806–810cmÀ1and$878cmÀ1as observed for the sul-fate–ferrihydrite samples(see Fig.1)and were attributed to the As–O stretching vibration of bidentate binuclear complexed arsenate ions.The formation of a ferric arse-nate surface precipitate at acidic pH was also evidenced by the change of the$3190cmÀ1O–H band with pH.The spectrum of ferrihydrite displayed strong NO3Àbands at1250–1500cmÀ1.These bands disappeared after adsorption of arsenate indicating that nitrate ions previ-ously adsorbed during ferrihydrite synthesis were dis-placed by arsenate ions.3.2.Effect of coverage density on the nature of adsorbed arsenateThe effect of coverage density on the nature of adsorbed arsenate on ferrihydrite was evaluated.At acidic pH(i.e. pH3,4),the As–O stretching vibration region(700–950cmÀ1)was dominated by a strong well-resolved band (Fig.5).As the Fe/As molar ratio increased from2to8(i.e.the adsorption density As/Fe decreasing from0.49to0.125)for the pH3sorption systems(see Table1),the intensity of the As–O stretching vibration band decreased markedly.The As–O stretching vibration peak was located at similar position.The pH4arsenate–ferrihydrite sorptionThe nature of adsorbed arsenate on ferrihydrite1649samples with various arsenate sorption densities exhibited very similar infrared spectra to that of pH3samples.Poor-ly crystalline ferric arsenate was the major surface arsenate species.The nature of surface arsenate species was not sig-nificantly influenced by arsenate sorption density and equi-librium concentration.At pH6,the major As–O stretching vibration peak was located at$822cmÀ1for all the initial Fe/As molar ratios used,i.e.2,4and8.A visible shoulder was also emerging at $878cmÀ1on the infrared spectra.A bidentate complex of arsenate was becoming detectable in addition to the major surface species of ferric arsenate.When pH increased to8,all infrared spectra exhib-ited two bands at808and878cmÀ1irrespective of the initial Fe/As molar ratios indicating that bidentate com-plexes were the dominant surface arsenate species.The equilibrium concentration of arsenic ranged from0.028 to15.8mM for the pH8sorption systems and the sorp-tion density(As/Fe)ranged from0.125to0.31.Waych-unas et al.(1993)conducted EXAFS analysis on samples with sorption density of0.001–0.1and concluded that arsenate was sorbed via bidentate surface complexation. Goldberg and Johnston(2001)used FTIR to character-ize the arsenate–iron oxide sorption solids synthesized at pH5and9with equilibrium concentration of0.1–1.0mM and suspension density of4g/L($8g/L for the present work).The obtained results showed similar As–O band to the present study,i.e.two bands located at$817–824and$854–861cmÀ1.The infrared spectrum obtained by Roddick-Lanzilotta et al.(2002)for the sorption system pH2.6and arsenic equilibrium concen-tration of$0.5mM displayed a well-resolved band $825,which is similar to the single As–O band of the acidic samples in this work.Carlson et al.(2002)pro-posed the formation of ferric hydroxyarsenate in Fe/As=2.6–3.2sorption solids prepared from solutions of pH3.Therefore,by comparing the present study with other works,it is proposed that the pH plays the most impor-tant role in controlling the nature of the surface arsenate species sorbed on ferrihydrite,whereas other conditions (e.g.coverage density,suspension density etc.)play less important factors.The fate of sulfate in synthetic ferrihydrite before and after adsorption of arsenate can also be monitored on the infrared spectra.As indicated by the bands between950 and1250cmÀ1,more sulfate was incorporated into the fer-rihydrite at acidic pH(3,4)than neutral to alkaline pH (6,8)(Fig.5).After adsorption of arsenate at pH3,4 and Fe/As=4,8,there was still measurable amount of sul-fate remaining in the ferrihydrite,while at Fe/As=2,all sulfate has been desorbed.It was proposed that sulfate ions were adsorbed on goethite as both inner-sphere and outer-sphere surface complexes at acidic pH(Peak et al.,1999). Adsorption of arsenate on the ferrihydrite involved ligand exchange with previously adsorbed sulfate ions.With increasing pH,the adsorbed sulfate ions on ferrihydrite were more easily displaceable by arsenate.At pH6,sulfate bands were visible only on the Fe/As=8infrared spectrum while no sulfate was detectable by FTIR in the pH8sam-ples.This is in good agreement with a previous study that reported the quantitative analysis of the sorption solids (Jia and Demopoulos,2005).It was suggested that surface precipitation of phosphate and arsenate on goethite occurred almost simultaneously (Zhao and Stanforth,2001;Ler and Stanforth,2003).The process of surface precipitation may involve slow dissolution of ferrihydrite,ternary complexation of Fe3+ and subsequent precipitating of arsenate(Ler and Stan-forth,2003).The process was depicted schematically in Fig.6.It was not surprising that arsenate surface precipitate formed at such a high initial Fe/As molar ratio of8where arsenic equilibrium concentration is below the detection limit(<0.02mg/L)in this work.There possibly also existed tridentate complex structure that formed initially upon contacting of the arsenate ions with ferrihy-drite given the amorphous nature of the latter.The saturation state with respect to poorly crystalline ferric arsenate in acidic media was estimated in a previous study(Jia et al.,2006).When the p K sp(ferrihydrite)=39 (Langmuir,1997)and p K sp(ferric arsenate)=22.89 (Mahoney,2002)were taken in the calculation,the log IAPð½Fe3þ ½AsO43À was obviously lower than log K sp (ferric arsenate)for the pH3,Fe/As=4and Fe/As=8 sorption systems,indicating that the systems were undersat-urated with respect to poorly crystalline ferric arsenate.For。
EN 71-11-2005
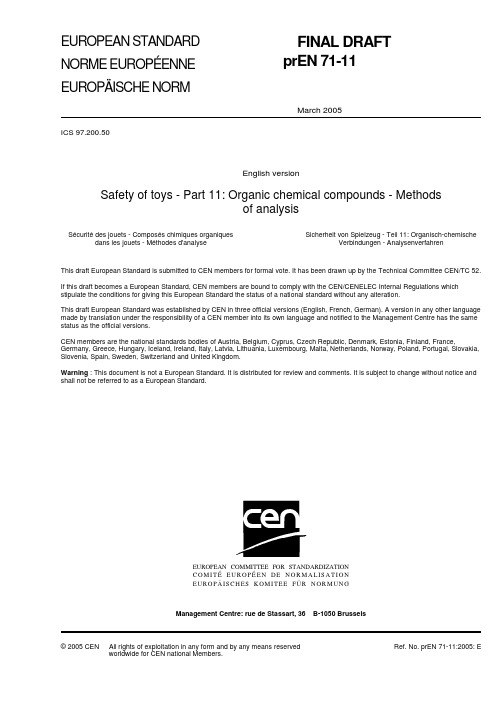
© 2005 CEN
All rights of exploitation in any form and by any means reserved worldwide for CEN national Members.
Ref. No. prEN 71-11:2005: E
prEN 71-11:2005 (E)
Contents
Page Foreword..............................................................................................................................................................3 Introduction .........................................................................................................................................................4 1 2 3 4 5 5.1 5.2 5.3 5.4 5.5 5.6 5.7 5.8 Scope ......................................................................................................................................................5 Normative references ............................................................................................................................5 Terms and definitions ...........................................................................................................................5 Environmental, health and safety precautions...................................................................................6 Methods of analysis ..............................................................................................................................6 General....................................................................................................................................................6 Flame retardants ....................................................................................................................................6 Colourants ..............................................................................................................................................9 Primary aromatic amines ....................................................................................................................15 Monomers and solvents......................................................................................................................18 Wood preservatives.............................................................................................................................36 Preservatives........................................................................................................................................40 Plasticisers ...........................................................................................................................................43
铜锌镁铝四元水滑石的微观结构及其姜-泰勒畸变
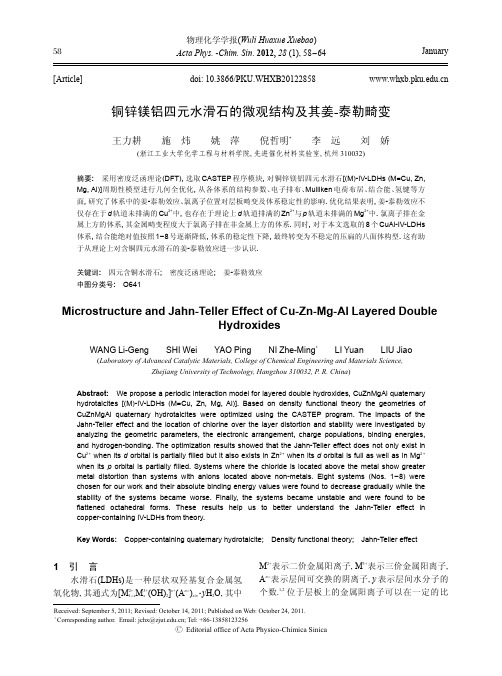
铜锌镁铝四元水滑石的微观结构及其姜-泰勒畸变王力耕施炜姚萍倪哲明*李远刘娇(浙江工业大学化学工程与材料学院,先进催化材料实验室,杭州310032)摘要:采用密度泛函理论(DFT),选取CASTEP 程序模块,对铜锌镁铝四元水滑石[(M)-IV-LDHs (M=Cu,Zn,Mg,Al)]周期性模型进行几何全优化,从各体系的结构参数、电子排布、Mulliken 电荷布居、结合能、氢键等方面,研究了体系中的姜-泰勒效应、氯离子位置对层板畸变及体系稳定性的影响.优化结果表明,姜-泰勒效应不仅存在于d 轨道未排满的Cu 2+中,也存在于理论上d 轨道排满的Zn 2+与p 轨道未排满的Mg 2+中.氯离子排在金属上方的体系,其金属畸变程度大于氯离子排在非金属上方的体系.同时,对于本文选取的8个CuAl-IV-LDHs 体系,结合能绝对值按照1-8号逐渐降低,体系的稳定性下降,最终转变为不稳定的压扁的八面体构型.这有助于从理论上对含铜四元水滑石的姜-泰勒效应进一步认识.关键词:四元含铜水滑石;密度泛函理论;姜-泰勒效应中图分类号:O641Microstructure and Jahn-Teller Effect of Cu-Zn-Mg-Al Layered DoubleHydroxidesWANG Li-GengSHI WeiYAO PingNI Zhe-Ming *LI YuanLIU Jiao(Laboratory of Advanced Catalytic Materials,College of Chemical Engineering and Materials Science,Zhejiang University of Technology,Hangzhou 310032,P .R.China )Abstract:We propose a periodic interaction model for layered double hydroxides,CuZnMgAl quaternary hydrotalcites [(M)-IV-LDHs (M=Cu,Zn,Mg,Al)].Based on density functional theory the geometries of CuZnMgAl quaternary hydrotalcites were optimized using the CASTEP program.The impacts of the Jahn-Teller effect and the location of chlorine over the layer distortion and stability were investigated by analyzing the geometric parameters,the electronic arrangement,charge populations,binding energies,and hydrogen-bonding.The optimization results showed that the Jahn-Teller effect does not only exist in Cu 2+when its d orbital is partially filled but it also exists in Zn 2+when its d orbital is full as well as in Mg 2+when its p orbital is partially filled.Systems where the chloride is located above the metal show greater metal distortion than systems with anions located above non-metals.Eight systems (Nos.1-8)were chosen for our work and their absolute binding energy values were found to decrease gradually while the stability of the systems became worse.Finally,the systems became unstable and were found to be flattened octahedral forms.These results help us to better understand the Jahn-Teller effect in copper-containing IV-LDHs from theory.Key Words:Copper-containing quaternary hydrotalcite;Density functional theory;Jahn-Teller effect[Article]doi:10.3866/PKU.WHXB20122858物理化学学报(Wuli Huaxue Xuebao )Acta Phys.-Chim.Sin .2012,28(1),58-64JanuaryReceived:September 5,2011;Revised:October 14,2011;Published on Web:October 24,2011.∗Corresponding author.Email:jchx@;Tel:+86-138********ⒸEditorial office of Acta Physico-Chimica Sinica1引言水滑石(LDHs)是一种层状双羟基复合金属氢氧化物,其通式为[M 2+1-x M 3+x (OH)2]x +(A n -)x /n·y H 2O,其中M 2+表示二价金属阳离子,M 3+表示三价金属阳离子,A n -表示层间可交换的阴离子,y 表示层间水分子的个数.1,2位于层板上的金属阳离子可以在一定的比58王力耕等:铜锌镁铝四元水滑石的微观结构及其姜-泰勒畸变No.1例范围内被离子半径相近的金属阳离子同晶取代,从而形成二元、三元、四元水滑石.目前,对于水滑石材料的理论模拟多为二元、三元结构,3-6而对四元水滑石的理论研究涉及较少.随着水滑石制备技术的进步及其应用领域的拓宽,多元水滑石材料也受到了广泛关注,冯拥军7和吴健松8等分别选用不同方法合成了CuNiMgAl 、CuZnMgAl 四元水滑石,并通过X 射线衍射(XRD)等表征方法证明了其晶型的完整性;Wang 等9发现CuZnMgAl 四元水滑石对加氢反应具有催化活性;张军等10采用共沉淀法合成了NiMgAlLa 四元水滑石,并将其应用于甲烷的部分氧化,性能优越,但从实验手段很难精确获得这些四元水滑石的微观结构、电子性质等特性.因此,计算机模拟技术的引入就显得尤为重要.密度泛函理论(DFT)11,12是一种研究多电子体系电子结构的量子力学方法,目前已被广泛应用于计算水滑石体系的结构参数、成键状况、作用能、电子密度以及了解分子间交互作用的电子性质等.Wei 等3以簇模型[M(OH 2)6]n +(M 为Ca 2+、Mg 2+、Cu 2+、Ga 3+等金属阳离子)为基础,采用混合密度泛函B3LYP 方法讨论了体系中的姜-泰勒效应对结构参数的影响,并在此基础上进一步比较了不同阳离子组成的水滑石的结构和性质.本课题组13曾采用密度泛函理论分析了铜锌铝三元水滑石(Cu x Zn 3-x Al-LDHs,x =0-3)周期性模型的结构参数、姜-泰勒效应、Mulliken 电荷布居、结合能和氢键,探究了体系的畸变情况和稳定性.考虑到铜是常见的催化活性组元,具有价格低廉、毒性低等优点,被广泛应用于各种催化反应中,如Glaser 偶联反应、14Heck 反应、15不对称Henry 合成、16糠醛加氢、17碳杂偶联反应18等.所以,研究含铜水滑石的微观结构具有重要的意义.基于以上原因,本文采用密度泛函理论,构建了一系列氯离子插层的铜锌镁铝四元水滑石模型,从结构参数、Mulliken 电荷布居、结合能、氢键等角度研究了铜锌镁铝四元水滑石的姜-泰勒效应及氯离子位置对层板畸变和体系稳定性的影响,为进一步设计和制备具有姜-泰勒效应的水滑石材料提供理论参考.2计算模型与方法本文以2H 堆积模式1构建了铜锌镁铝四元水滑石的主体层板[Cu 2Zn 2Mg 2Al 2(OH)16]2+,层间氯离子可以排布在层板的Top 位、Hcp 位、Fcc 位以及Bridge 位(见图1(A)),氯离子总共在层板表面存在16种结合位置(见图1(B)).本课题组先前工作19表明氯离子位于Bridge 位和Top 位的构型都不能够稳定存在.因此,本文中只考虑氯离子位于Hcp 位和Fcc 位时的8种情况,共搭建了48种金属离子或氯离子不同排布的铜锌镁铝四元水滑石.采用先前工作中证实对水滑石体系适用的计算方法,19,20细节为:选用CASTEP 程序模块,21在LDA-CA-PZ 基组22水平对模型进行几何全优化,原子电子采用超软赝势,23截止能量为330.0eV ,自洽场计算的误差为2×10-6eV ·atom -1,能带结构在布里渊区k 矢量的选取为4×4×1,基态能量选用Pulay 密度混合算法,24整体电荷数为0,同时优化晶胞,其它参数设置为程序的默认值.优化结果表明,在48个铜锌镁铝四元水滑石模型中,Cu 在晶胞顶点,Al 在晶胞中心的8种模型能量相对较低,记作CuAl-IV-LDHs.本文即选取如图2所示的Cu 在顶点,Al 在中心,氯离子不同排布的8种图1Cl -在(M)-IV-LDHs (M=Cu,Zn,Mg,Al)中的几种不同位置Fig.1Models of (M)-IV-LDHs (M=Cu,Zn,Mg,Al)with different positions of Cl-(A)(B)59Acta Phys.-Chim.Sin.2012Vol.28情况进行分析.3结果与讨论3.1结合能分析为估计体系结构的稳定性,现定义CuAl-IV-LDHs体系的结合能ΔE CuAl-IV-LDHs为:ΔE CuAl-IV-LDHs=E CuAl-IV-LDHs-(16E H+16E O+2E Cl+2E Cu+2E Zn+2E Mg+2E Al)其中E CuAl-IV-LDHs为优化后CuAl-IV-LDHs体系的能量, E H=-1169.2kJ·mol-1、E O=-41364.3kJ·mol-1、E Cl=-39180.6kJ·mol-1、E Cu=-129759.8kJ·mol-1、E Zn=-165066.0kJ·mol-1、E Mg=-93770.7kJ·mol-1、E Al=-5114.7kJ·mol-1,为各原子的能量,结果列在表1中.从表1中可以看出,对于本文选取的CuAl-IV-LDHs体系,层间氯离子排布不同会影响其结合能, 1号到8号体系,结合能绝对值逐渐降低,体系的稳定性下降.氯离子在非Mg原子正上方,即CuAl-FccCuAlZn最稳定;氯离子在Mg原子正上方,即CuAl-HcpMg最不稳定.3.2结构参数分析将几何优化得到的CuAl-IV-LDHs的结构参数列入表2,晶胞参数a值和b值的大小主要归因于层板金属离子半径的影响.2即在CuAl-IV-LDHs体系中,主要是与晶胞棱上的Cu-O/Zn-O/Mg-O/ Al-O键长的大小相关.从表2可知,对于单独的CuAl-IV-LDHs层板(层间无氯离子时,即表2中Layer,下列各表中Layer均指单独的CuAl-IV-LDHs 层板),a=0.59003nm,b=0.59442nm.当氯离子插入层板以后,a、b值略微上升,这可能是由于当氯离表1CuAl-IV-LDHs的结合能Table1Binding energy of CuAl-IV-LDHsNo.1 2 3 4 5 6 7 8SystemCuAl-FccCuAlZnCuAl-HcpAlCuAl-FccMgAlCuCuAl-FccMgAlZnCuAl-HcpCuCuAl-HcpZnCuAl-FccCuZnMgCuAl-HcpMgE CuAl-IV-LDHs(kJ·mol-1)-1569771.8-1569757.0-1569753.4-1569752.2-1569742.3-1569724.0-1569697.7-1569696.1ΔE CuAl-IV-LDHs(kJ·mol-1)-23452.2-23437.4-23433.8-23432.6-23422.7-23404.4-23378.1-23376.5表2CuAl-IV-LDHs的晶胞参数Table2Lattice parameters of CuAl-IV-LDHsNo.12345678SystemLayerCuAl-FccCuAlZnCuAl-HcpAlCuAl-FccMgAlCuCuAl-FccMgAlZnCuAl-HcpCuCuAl-HcpZnCuAl-FccCuZnMgCuAl-HcpMga/nm0.590030.611820.611270.600210.599970.612070.619850.599930.61978b/nm0.594420.598360.600700.611790.609740.601000.601790.607680.60194c/nm1.475501.441251.478271.482861.486461.450741.456791.507461.53055图2CuAl-IV-LDHs模型Fig.2Models ofCuAl-IV-LDHs60王力耕等:铜锌镁铝四元水滑石的微观结构及其姜-泰勒畸变No.1子插入层板以后,Cu-O、Al-O、Mg-O、Zn-O的成键布居总体均降低,分别由单独层板的0.236e、0.382e、-1.145e、0.231e降至0.228e、0.374e、-0.973e、0.223e,使得金属-氧键键能下降,从而导致金属-氧键键长增大,a、b值略微上升.晶胞参数c值(层间距d c=0.5c)不仅受到层板金属离子半径的影响,还受到主客体间作用力大小的影响,2即晶胞参数c值为层板厚度和层间通道大小的加和的2倍.从表2中可知,1-8号体系的晶胞参数c值有增大的趋势,这主要是由于氯离子插入层板后,1-8号体系的结合能逐渐降低(数据见表1),说明体系的主客体作用力逐渐下降,从而使得层间通道增大,c值呈增大趋势.3.3Jahn-Teller效应分析1937年,姜(Jahn,H.A.)和泰勒(Teller,E.)指出:在对称的非线性分子中,如果有一个体系的基态有几个简并能级,则是不稳定的,体系会发生畸变,使能级发生改变,以消除简并性,这就是姜-泰勒效应.25水滑石层板类似于水镁石Mg(OH)2结构,它是由MO6八面体共用棱边所形成的,当其层板上的镁被铜等一些金属替换时,水滑石就会由于姜-泰勒效应发生畸变.对于CuAl-IV-LDHs体系中的Cu2+,d轨道电子数理论上为9,则有可能失去dz2或dx2-y2上的部分电子.若失去的是dz2上的电子,则会变成压扁的八面体构型;若失去的是dx2-y2上的电子,则会变成拉长的八面体构型.25结合表3中Cu-O键键长数据可知,1号体系中的6个Cu-O键出现了四个共面的短键和两个与该面垂直的长键,为典型的拉长的八面体构型,随着氯离子放置位置的变化,从1号到8号体系,短键基本上逐渐拉长,长键逐渐缩短,最终出现了四个共面的长键和两个与该面垂直的短键,为典型的压扁的八面体构型,说明1号到8号体系随着层间氯离子位置的改变,层板上的铜由易失去dx2-y2上的电子,逐渐转变为易失去dz2上的电子,体系的稳定性逐渐下降,这一现象与结合能分析结果一致.实验证明,Cu的六配位配合物,以拉长的八面体构型稳定存在,这是因为在无其它能量因素影响时,形成两条长键四条短键比形成两条短键四条长键的总键能要大的缘故.25表4中列出了CuAl-IV-LDHs体系中的原子轨道布居.从表4可以看出,体系中Zn2+的d轨道电子数为9.990e,相比Cu2+的d轨道电子数(9.425e-9.440e)更接近于饱和的d10状态,即Zn-O八面体配合物理论上不应该出现畸变现象.但从表5数据可以看出,在CuAl-IV-LDHs体系中,金属平均畸变角(指12个θOMO与理想六配位角度90°的绝对差值的平均No.1 2 3 4 5 6 7 8SystemLayerCuAl-FccCuAlZnCuAl-HcpAlCuAl-FccMgAlCuCuAl-FccMgAlZnCuAl-HcpCuCuAl-HcpZnCuAl-FccCuZnMgCuAl-HcpMgl Cu-O1/nm0.190030.190360.191290.191510.191250.190650.191870.190020.18904l Cu-O2/nm0.190280.190630.191510.191750.191320.191170.192060.190170.18916l Cu-O3/nm0.196440.198600.197880.198180.198770.200180.200320.203660.21571l Cu-O4/nm0.196460.198950.197970.198340.198950.200310.200410.203770.21572l Cu-O5/nm0.226240.234620.233580.233430.233300.230250.230640.228530.21704l Cu-O6/nm0.226300.234690.234870.233970.234030.232060.230800.228970.21819表3CuAl-IV-LDHs中的Cu-O键长Table3Cu-O bond lengths in CuAl-IV-LDHsNo.1 2 3 4 5 6 7 8SystemLayerCuAl-FccCuAlZnCuAl-HcpAlCuAl-FccMgAlCuCuAl-FccMgAlZnCuAl-HcpCuCuAl-HcpZnCuAl-FccCuZnMgCuAl-HcpMgQ(Al-p)/e0.8900.8900.8900.9000.8900.9000.9000.9300.900Q(Mg-p)/e5.5705.7705.7105.6705.7005.7205.7255.6705.610Q(Cu-p)/e0.4100.4200.4200.4000.4200.3900.4400.4100.450Q(Cu-d)/e9.4109.4309.4309.4309.4259.4409.4309.4309.430Q(Zn-p)/e0.6500.6500.6600.6800.6600.6700.6300.6500.660Q(Zn-d)/e9.9909.9909.9909.9909.9909.9909.9909.9909.990表4CuAl-IV-LDHs中的原子轨道布居Table4Atomic orbital populatins in CuAl-IV-LDHs61Acta Phys.-Chim.Sin.2012Vol.28值)ΔθOZnO值却大于ΔθOCuO值,在Zn-O八面体中也观察到了姜-泰勒畸变.同时,体系中没有d轨道电子的镁、铝配合物也存在一定程度的金属畸变,且ΔθOMgO值仅略低于ΔθOCuO值.据此可推测,不仅只有d轨道电子的不均匀排布会发生姜-泰勒畸变,镁的p轨道电子的不均匀排布也存在发生姜-泰勒畸变的可能性,因为只要p x、p y、p z中任一轨道上的电子缺失或者多余,导致三个轨道上的电子排布不相同,相应轴上的M-O键就会缩短或者拉长,从而导致畸变的产生.图3为CuAl-IV-LDHs体系平均金属畸变角的分布情况,总体上来说,氯离子排在金属上方的体系(2、5、6、8号体系)其金属八面体畸变程度大于氯离子排在非金属上方的体系,故氯离子排在非金属上方更有利于体系的稳定性.且从表5数据可知,1号到8号体系的平均金属畸变基本呈逐渐增大趋势,说明层板上的金属八面体畸变越来越严重.3.4Mulliken电荷布居分析Mulliken布居是Mulliken26提出的表示电子在各原子轨道上分布情况的方法,它可以间接地讨论分子内相互作用力的强弱,尤其对同一系列的分子十分奏效.因此,为了进一步研究CuAl-IV-LDHs体系的作用力,对其进行了电子分析,得到的Mulliken 电荷布居(对上下的层板、客体氯离子、各原子及键的电荷布居作了平均处理)列入表6和表7中.从表6、7可以看出,当CuAl-IV-LDHs体系中插入氯离子后,Cu、Zn、Mg、Al所带的电荷数均减少,说明氯离子的电荷向层板发生了转移,转移的数量大小顺序为Cu>Zn>Al>Mg,层板与层间氯离子的静电作用增强.且Cu-O、Al-O、Mg-O、Zn-O键布居的绝对值也基本上减少,故层板上M-O键的离子键作用力亦减弱,说明氯离子的引入,虽增加表5CuAl-IV-LDHs中的金属畸变角Table5Metal distortion angle of CuAl-IV-LDHsNo.1 2 3 4 5 6 7 8SystemLayerCuAl-FccCuAlZnCuAl-HcpAlCuAl-FccMgAlCuCuAl-FccMgAlZnCuAl-HcpCuCuAl-HcpZnCuAl-FccCuZnMgCuAl-HcpMgAverage distortion of metal/(°)ΔθOMgO/(°)6.60810.0997.6567.4247.7789.8868.2497.7639.2228.298ΔθOZnO/(°)8.8907.65310.08210.50010.2377.9819.7989.8518.7859.309ΔθOCuO/(°)7.6708.6249.0768.8108.9859.0989.0928.8529.1738.820ΔθOAlO/(°)3.2623.9033.6333.8123.5644.3334.1874.8224.2383.973Average metal distortion/(°)6.6087.5707.6127.6377.6417.8257.8327.8227.855ΔθOMO:systemʹs average metal distortion angle图3CuAl-IV-LDHs平均金属畸变情况Fig.3Average metal distortion ofCuAl-IV-LDHsNo.12345678SystemLayerCuAl-FccCuAlZnCuAl-HcpAlCuAl-FccMgAlCuCuAl-FccMgAlZnCuAl-HcpCuCuAl-HcpZnCuAl-FccCuZnMgCuAl-HcpMgQ(Cu-O)/e0.2360.2370.2300.2280.2230.2170.2270.2270.228Q(Al-O)/e0.3820.3740.3720.3680.3660.3700.3720.3700.374Q(Mg-O)/e-1.145-1.013-1.023-0.992-0.968-0.956-0.966-0.963-0.973Q(Zn-O)/e0.2310.2380.2270.2250.2290.2290.2140.2120.223Q(H-O)/e0.6100.5860.5880.5950.5900.6000.5900.6040.606表6CuAl-IV-LDHs的Mulliken成键布居Table6Mulliken bond population of CuAl-IV-LDHs62王力耕等:铜锌镁铝四元水滑石的微观结构及其姜-泰勒畸变No.1了主客体的作用力,但会相对地削弱层板自身的作用力.同时,纵向比较,从1号到8号体系,Cl -所带的负电荷呈增大趋势,说明从客体向主体层板转移的电子呈下降趋势,整体层板电荷增大,说明随着体系稳定性的减弱,主客体的静电作用力呈增强趋势,而层板自身的成键作用减弱.3.5CuAl-IV-LDHs 的氢键结构分析氢键会对材料的微观结构造成较大的影响,它是一种广泛存在的分子间弱作用力,是特殊的分子间或分子内作用.它是由极性很强的X -H 键上的氢原子与另一个键(可存在于同一种分子或另一种分子中)上电负性很强、原子半径较小的Y 原子(如F 、N 、O 等)的孤对电子之间相互吸引而形成的一种键(用X -H …Y 表示).27-29一般情况下,氢键具有方向性和饱和性,氢键的键长越短、键角越接近于180°,氢键的强度越强.铜锌镁铝四元水滑石体系中存在多重的氢键,它与一般的氢键性质不同,层间氯离子并不只与层板上的一个羟基中的氢原子形成氢键,而是与多个羟基上的氢原子形成多重氢键.本文选取的CuAl-IV-LDHs 体系的氢键数据列于表8和图4中.由表8和图4可见,氯离子排在非金属上方的体系氢键强度强于氯离子排在金属上方的体系.故氯离子排在非金属上方更有利于体系的稳定性.此外,1号体系CuAl-FccCuAlZn 的单个氢键的强度较大,且共形成了12个氢键,故其强度较大;而8号体系CuAl-HcpMg 不管是形成的单个氢键的强度还是氢键数目,都是不利于体系的稳定性的.总体上来说,从1号到8号体系,氢键强度逐渐减弱.4结论本文采用赝势平面波法CASTEP 计算了(M)-IV-LDHs (M=Cu,Zn,Mg,Al)的结构和能量,选取了表7CuAl-IV-LDHs 的Mulliken 电荷布居Table 7Mulliken charge population of CuAl-IV-LDHsNo.12345678System LayerCuAl-FccCuAlZn CuAl-HcpAlCuAl-FccMgAlCu CuAl-FccMgAlZn CuAl-HcpCu CuAl-HcpZnCuAl-FccCuZnMg CuAl-HcpMg Q (H)/e 0.4010.3880.3860.3860.3880.3880.3910.3910.390Q (O)/e -0.952-0.949-0.952-0.953-0.954-0.953-0.952-0.955-0.958Q (Mg)/e 2.1201.9101.9701.9981.9551.9501.9752.0102.055Q (Al)/e 1.5801.5501.5601.5601.5601.5701.5601.5701.565Q (Zn)/e 1.0400.9700.9600.9700.9700.9900.9900.9900.980Q (Cu)/e 0.6700.6150.6200.6400.6500.6300.6500.6400.650Q (Layer)/e-0.5570.5820.6320.6070.620.6870.6980.671Q (Cl)/e --0.590-0.600-0.635-0.620-0.630-0.685-0.690-0.685表8CuAl-IV-LDHs 的氢键结构参数Table 8Hydrogen bond parameters of CuAl-IV-LDHsNo.12345678System CuAl-FccCuAlZn CuAl-HcpAlCuAl-FccMgAlCu CuAl-FccMgAlZn CuAl-HcpCu CuAl-HcpZnCuAl-FccCuZnMg CuAl-HcpMgBond length/nm0.227700.228910.223600.233060.230510.226310.232900.24106Charge/e 0.0650.0600.0700.0580.0570.0650.0610.048Number of hydrogen bonds1212812128811Bond angle/(°)154.951150.791153.026151.452152.698151.330146.558144.939图4CuAl-IV-LDHs 氢键键长和电荷关系图Fig.4Relation of charges and hydrogen bond lengths ofCuAl-IV-LDHsThe number x (y )in the figure means that there are y hydrogen bonds for the system x .refers to anion on the nonmetal atoms andrefers to anion on the metalatoms.63Acta Phys.-Chim.Sin.2012Vol.28能量相对较低的CuAl-IV-LDHs体系,探讨了体系的姜-泰勒效应以及氯离子位置对层板畸变的影响,结论如下:(1)对于CuAl-IV-LDHs体系,姜-泰勒效应不仅存在于d轨道未排满的Cu2+中,在p轨道未排满的Mg2+、Al3+中也可能存在.对于1号到8号体系的平均金属畸变呈逐渐增大趋势,说明层板上的金属八面体畸变越来越严重,不利于层板中金属八面体的稳定性.(2)层板中的铜原子与六个氧原子配位,从1号到8号体系,六个Cu-O键先出现四长键二短键的分化,为拉长的稳定的八面体,再逐渐发生构型转变,最后转变为四短键二长键的压扁的不稳定的八面体形式.(3)通过对CuAl-IV-LDHs体系的氢键、结合能的分析,氯离子排在非金属上方的体系氢键强度强于氯离子排在金属上方的体系,故氯离子排在非金属上方更有利于体系的稳定性.对于1号到8号体系,总结合能绝对值逐渐降低,体系稳定性下降,氯离子在非Mg原子正上方,即CuAl-FccCuAlZn最稳定;氯离子在Mg原子正上方,即CuAl-HcpMg最不稳定.References(1)Cavani,F.;Trifiro,F.;Vaccari,A.Catal.Today1991,11,173.(2)Duan,X.;Zhang,F.Z.Intercalation and Assembly Chemistry ofInorganic Supramolecular Materials;Science Press:Beijing,2009.[段雪,张法智.无机超分子材料的插层组装化学.北京:科学出版社,2009.](3)Yan,H.;Lu,J.;Wei,M.;Ma,J.;Li,H.;He,J.;Evans,D.G.;Duan,X.J.Mol.Struct.-Theochem2008,866,34.(4)Becke,A.D.Chem.Phys.1993,98,5648.(5)Lee,C.;Yang,W.;Parr,R.G.Phys.Rev.B1988,37,785.(6)Yan,H.;Wei,M.;Ma,J.;Li,F.;Evans,D.G.;Duan,X.J.Phys.Chem.A2009,113,6133.(7)Feng,Y.J.;Li,D.Q.;Li,C.X.;Wang,Z.G.Acta ChimicaSinica2003,61,78.[冯拥军,李殿卿,李春喜,王子镐.化学学报,2003,61,78.](8)Wu,J.S.;Xiao,Y.K.;Lin,Y.P.;Liang,H.Q.;Li,C.Y.;He,H.Y.Journal of Synthetic Crystals2010,39,817.[吴健松,肖应凯,林意萍,梁海群,李春银,何海英.人工晶体学报,2010,39,817.](9)Wang,X.P.;Yu,J.J.;Cheng,J.;Hao,P.Z.;Xu,P.Z.Environ.Sci.Technol.2008,42,614.(10)Zhang,J.;Zhao,N.;Wei,W.;Sun,Y.H.The Research on UsingQuaternary Hydrotalcites for the Partial Qxidation of Methane.In The Proceedings of The Seventh National Conference onCatalysis Science and Technology,The Seventh NationalConference on Catalysis Science and Technology,Taiyuan,2009;pp319-321.[张军,赵宁,魏伟,孙予罕.新型四元水滑石在甲烷部分氧化中的应用研究.第七届全国催化剂制备科学与技术研讨会会议论文集,第七届全国催化剂制备科学与技术研讨会,太原,2009:319-321.](11)Heermann,puter Simulation Methods in TheoreticalPhysics;Springer-Verlag Press:Heidelberg,1990;pp387-439.(12)Leach,A.R.Molecular Modelling:Principles and Applications;Addison Wesley Longman Limitted Press:Essex,2001;pp26-454.(13)Ni,Z.M.;Yao,P.;Liu,X.M.;Wang,Q.Q.;Xu,Q.Chem.J.Chin.Univ.2010,31,2438.[倪哲明,姚萍,刘晓明,王巧巧,胥倩.高等学校化学学报,2010,31,2438.](14)Tang,J.Y.;Jiang,H.F.;Deng,G.H.;Zhou,.Chem.2005,25,1503.[唐金玉,江焕峰,邓国华,周磊.有机化学,2005,25,1503.](15)Suresh,I.;Vinay,V.T.J.Mol.Catal.A:Chem.2000,157,275.(16)Matthew,D.J.;Christine,J.C.;Mary,F.M.;Paul,R.R.;David,A.;Joann,W.;David,C.J.Mol.Catal.A:Chem.2010,325,8.(17)Surapas,E.R.Catal.Lett.2011,141,784.(18)Xu,J.H.;Man,Q.S.;Lin,Y.C.;Li,Y.Y.;Feng,Y.S.Chin.J.Org.Chem.2010,30,9.[许建华,蔄秋实,林义成,李源源,冯乙巳.有机化学,2010,30,9.](19)Xu,Q.;Ni,Z.M.;Mao,J.H.J.Mol.Struct:Theochem.2009,915,122.(20)Xu,Q.;Ni,Z.M.;Pan,G.X.;Chen,L.T.;Liu,T.ActaPhys.-Chim.Sin.2008,24,601.[胥倩,倪哲明,潘国祥,陈丽涛,刘婷.物理化学学报,2008,24,601.](21)Segall,M.D.;Linda,P.;Probert,M.;Pickard,C.;Hasnip,P.;Clark,S.;Payne,M.J.Phys.-Condes.Matter2002,14,2717.(22)Ceperley,D.M.;Aider,B.J.Phys.Rev.Lett.1980,45,566.(23)Vanderbilt,D.Phys.Rev.B1990,41,7892.(24)Kresse,G.;Furthmiiller,J.Phys.Rev.B1996,54,11169.(25)Pan,D.K.;Zhao,C.D.;Zheng,Z.X.The Structure of Matter;Higher Education Press:Beijing,1989;pp329-330.[潘道皑,赵成大,郑载兴.物质结构.北京:高等教育出版社,1989:329-330.](26)Mulliken,R.S.J.Chem.Phys.1955,23,1833.(27)Scheiner,S.Hydrogen Bonding;Oxford University Press:NewYork,1997.(28)Jeffrey,G.A.An Introduction to Hydrogen Bond;OxfordUniversity Press:New York,1997.(29)Desiraju,G.;Steiner,T.The Weak Hydrogen Bond;OxfordUniversity Press:New York,1999.64。
文献信息检索教程期末考试题库含大量真题

《大学生信息检索概论》模拟试题一、填空题1、文献的级次分为零次文献、一次文献、二次文献、三次文献2、《中图法》有五个基本部类,分别是马克思主义、列宁主义、毛泽东思想_、哲学;社会科学;自然科学和综合性图书,在此基础上又划分为_22_个大类。
3、按内容可将计算机检索系统的数据库类型分为:文献书目型数据库、事实型数据库、数值型数据库和全文型数据库。
4、我国标准可分为国家标准、部标准和企业标准三大类。
5、在实际检索中,文献的检索方法主要有:直查法、追溯法、工具法和综合法。
6二、选择题1、如果需要检索某位作者的文献被引用的情况,应该使用( C )检索。
A.分类索引 B.作者索引 C.引文索引 D.主题索引2、利用图书馆的据库检索期刊论文时,可供选择的中文数据库是( D )。
A.超星数字图书馆 B.万方学位论文 C.国研网 D.维普科技期刊 E.高校财经库3、如果检索有关多媒体网络传播方面的文献,检索式为(A D)。
A.多媒体and 网络传播 B.多媒体+网络传播 C.多媒体or 网络传播 D.多媒体*网络传播4。
A.关键词 B.作者 C.刊名 D.题名 E.文摘5、二次文献又称检索工具,包括:。
A.书目 B.百科 C.索引 D.文摘 E.统计数据三、名词解释题1、文献2、体系分类语言3、引文语言4、事实检索5、二次文献四、问答题1、简述论文选题的基本原则?2、制定检索策略的步骤与技巧是什么?3、通过本课程的学习,你有哪些收获和体会?(发挥题)一、单项选择题(请将正确答案的序号填在括号内,每题分,共30分)1. )A 载体B 纸张C 光盘D 磁盘2. 下列哪种文献属于一次文献A 期刊论文B 百科全书C 综述D 文摘3. 下列哪种文献属于二次文献A 专利文献B 学位论文C 会议文献D 目录4. 下列哪种文献属于三次文献( C )A 标准文献B 学位论文C 综述D 文摘5. 下列选项中属于连续出版物类型的选项有( C )A 图书B 学位论文C 科技期刊D 会议文献6. 下列选项中属于特种文献类型的有( D )A 报纸B 图书C 科技期刊D 标准文献7. 纸质信息源的载体是( D )A 光盘B 缩微平片C 感光材料D 纸张8. 以刊载新闻和评论为主的文献是( B )A 图书B 报纸C 期刊D 会议文献9. 使用分类语言对信息进行描述和标引,主要是可以把( B )的信息集中在一起。
燃料电池专业词汇
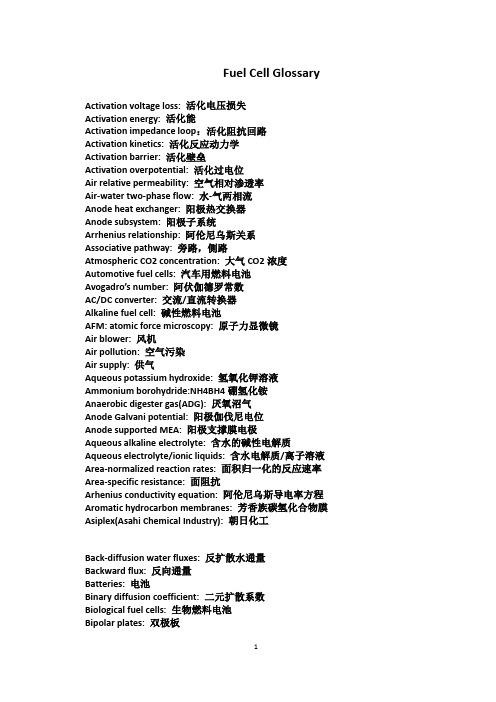
Fuel Cell Glossary Activation voltage loss: 活化电压损失Activation energy: 活化能Activation impedance loop:活化阻抗回路Activation kinetics: 活化反应动力学Activation barrier: 活化壁垒Activation overpotential: 活化过电位Air relative permeability: 空气相对渗透率Air-water two-phase flow: 水-气两相流Anode heat exchanger: 阳极热交换器Anode subsystem: 阳极子系统Arrhenius relationship: 阿伦尼乌斯关系Associative pathway: 旁路,侧路Atmospheric CO2 concentration: 大气CO2浓度Automotive fuel cells: 汽车用燃料电池Avogadro’s number: 阿伏伽德罗常数AC/DC converter: 交流/直流转换器Alkaline fuel cell: 碱性燃料电池AFM: atomic force microscopy: 原子力显微镜Air blower: 风机Air pollution: 空气污染Air supply: 供气Aqueous potassium hydroxide: 氢氧化钾溶液Ammonium borohydride:NH4BH4硼氢化铵Anaerobic digester gas(ADG): 厌氧沼气Anode Galvani potential: 阳极伽伐尼电位Anode supported MEA: 阳极支撑膜电极Aqueous alkaline electrolyte: 含水的碱性电解质Aqueous electrolyte/ionic liquids: 含水电解质/离子溶液Area-normalized reaction rates: 面积归一化的反应速率Area-specific resistance: 面阻抗Arhenius conductivity equation: 阿伦尼乌斯导电率方程Aromatic hydrocarbon membranes: 芳香族碳氢化合物膜Asiplex(Asahi Chemical Industry): 朝日化工Back-diffusion water fluxes: 反扩散水通量Backward flux: 反向通量Batteries: 电池Binary diffusion coefficient: 二元扩散系数Biological fuel cells: 生物燃料电池Bipolar plates: 双极板Blocking electrode: 阻断电极Boltzmann’s constant: 波尔兹蔓常数Bonding energy: 键能Bottleneck processes: 瓶颈技术Boundary conditions: 边界条件Boundary layer theory: 边界层理论Bonding energy: 键能Built-in voltage: 内置电压Bulk reactant: 总体反应物Butler-Volmer equation: 巴特勒-沃尔默方程Bypass valve: 旁通阀BYZ:Yttrium doped barium zirconate:钇掺杂的锆酸钡Bruggeman correlation: 布鲁格曼相关系数Capacitive double-layer impedance: 电容式双层阻抗Capacitors: 电容器Carbon cloth(paper): 碳布(纸)Carbon dioxide: 二氧化碳Carbon monoxide tolerance: 一氧化碳耐受度Carbon emission: 碳排放Carnot cycle: 卡诺循环Carrier-gas based fuel-processing subsystem: 载气燃料处理系统Carrier hydrogen storage system: 载波储氢系统Carriers concentration:载流浓度Catalyst layer: 催化层Catalyst layer interface: 催化层界面Catalyst reactant: 催化反应物Catalyst deactivation: 催化剂失活Catalytic electrode: 催化电极Catalytic fuel reformer: 催化燃料重整器Cathode Galvani potential: 阴极伽伐尼电位Cathode-membrane interface: 阴极-膜界面Cathode subsystem: 阴极子系统Cathode intercooler: 阴极中冷器Cathode degradation: 阴极衰减Cathode intercooler: 阴极中冷器Cathode activation overvoltage: 阴极活化过电位Cathode supported MEA: 阴极支撑的膜电极Ceramic electrolytes: 陶瓷电解质Ceramic materials: 陶瓷材料Chemisorptions: 化学吸附Current density: 电流密度Cell voltage variation: 电池电压变化Cluster-network model: 串网络模型Cold-start cell voltage: 冷启动电池电压Ceria-based anodes: 铈基阳极CFD: computational fluid dynamics: 计算流体动力学Charged species: 带电粒子Charge flux: 电荷通量Charge carriers: 电荷载体Charge conservation: 电荷守恒Charge transfer mechanisms: 电荷传输机理Charge transfer reactions: 电荷传输反应Charge transport: 电荷传输Chemical bonds: 化学键Chemical determinations: 化学测定法Chemical energy: 化学能Chemical free energy difference: 化学自由能差Chemical potential: 化学电位Chemical reaction: 化学反应Chemical surface oxidation: 化学表面氧化Chemical Structure:化学结构Chemisorbed hydrogen charge transfer reaction: 基于化学吸附的氢气传输反应CHP: combined heat and power: 热电联产(供)Chromium-based metallic interconnects: 铬基金属连接Circuit diagram: 电路图Climate change: 气候变化Climate mitigation: 减缓气候变化Closed system: 封闭系统Coal gasification plant: 煤气化工厂Cold stream:冷流Compressed hydrogen/air: 压缩氢气/空气Compression force: 压缩力Composite membranes: 复合膜Concentration gradient: 浓度梯度Concentration losses: 浓度损失Condenser heat: 冷凝热Conductivity: 导电率Conservation laws: 守恒定律Conservation equations: 守恒方程Constant-current-density assumption: 恒电流密度假设Constant flow rate condition: 流量恒定条件Constant and variable-flow models: 恒流-变流模型Constant-pressure process: 恒压过程Constant stoichiometry: 恒定化学计量比Constitutive equations:本构方程Continuity equation: 连续性方程Continuum diffusion coefficient: 连续性扩散系数Control volume method: 体积控制法Consumption rate: 消耗速率Contact resistance: 接触电阻Convection diffusion: 对流扩散Convective-dominated flow: 对流控制流体Convective fluid transport: 对流流体传输Convective forces: 对流力Convective heat transfer: 对流换热Convective heat-transfer coefficient: 对流传热系数Convective mass transport process: 对流传质过程Convective transport: 对流传输Cooling requirement: 冷却条件Cooling systems: 冷却系统Coupling coefficients: 耦合系数Coupled thermal-water management: 耦合的水热管理Crystal structure: 晶体结构Cross-membrane interconnections: 膜间互连结构Cross section: 断面Crystalline electrolyte: 晶体电解质Current interrupt technique: 电流中断技术Current-voltage curve: 电流-电压曲线Cyclic voltammetry: 循环伏安(法)Capillary flux: 毛细通量Darcy’s law: 达西定律Derivation: 衍生,推导Diffusion coefficients: 扩散系数Diffusion mechanism: 扩散机理Diffusion transport: 扩散传输Diffusivity: 扩散系数Diffusion flux: 扩散通量Diffusion Layers:扩散层Dimensionless groups: 无量纲组Dimensionless factor: 无量纲因子Dissociative pathway: 分解途径Dual-layer cathode electrode: 双层阴极Dusty-fluid model: 尘流模型DC-DC converter: 直流-直流转换器Dead zones: 盲端Decay rate: 衰减速率Dependent variables: 独立变量Dielectric breakdown: 介电击穿Dilute solution(ideal solution): 稀溶液(理想溶液)Direct alcohol fuel cells: 直接醇燃料电池Direct electro-oxidation: 直接电氧化Direct formic acid fuel cells: 直接甲酸燃料电池Direct methanol fuel cells: 直接甲醇燃料电池Dopant ions: 掺杂离子Doped carbon: 碳掺杂Double-layer capacitance: 双层电容Dual-layer approach: 双层接触Durability: 耐久性Dynamic equilibrium: 动态平衡Economic trade-off: 经济权衡(效益/性价比)Electro-osmotic flow: 电渗流Electrochemical reaction kinetics: 电化学反应动力学Electrode-electrolyte interface: 电极-电解质界面Electrolyte membrane: 电解质膜Electromotive force: 电动势Empirical correlation: 经验相关性Energy balance: 能量平衡Enthalpy change: 焓变Entropic heat: 熵热Effective diffusivity: 有效扩散Effective porosity: 有效孔隙率Effective resistance: 有效阻抗Efficiency loss: 效率损失EIS: electrochemical impedance spectroscopy:电化学阻抗谱图分析Electrochemical reaction kinetics: 电化学反应动力学Electrical efficiencies: 发电效率Electrical driving forces: 电子驱动力Electrical potential: 电压Electrical field force: 电场力Electric power: 电功Electric wall conditions: 电壁条件Electrical work: 电功Electrochemical half reactions: 电化学半反应Electrochemical reaction heterogeneity: 电化学反应异质性Electrode-electrolyte microstructure: 电极-电解质微观结构Electrode materials: 电极材料Electro-osmotic drag: 电渗拖拽Endothermic reaction: 吸热反应Exothermic reaction: 放热反应Energy buffers: 能量缓冲器Energy conversion device: 能量转换装置Equivalent circuit elements: 等效电路元件Equivalent circuit (fuel cell) model: 等效电路(燃料电池)模型Equivalent weight: 当量Exponential Boltzmann factor: 指数波尔兹曼因子External heat transfer: 外部传热Extrinsic carriers: 外源载体Exchange current: 交换电流Experimental visualization: 可视化实验Extrinsic carriers: 外源载体Faraday’s law: 法拉第定律Fick’s law: 费克定律Fickian diffusion (coefficients): 费克扩散系数First law of thermodynamics: 热力学第一定律Flooding: 水淹Flow equations: 流量方程Fluid mixer: 混合流、流体混合器Four-point membrane –conductivity measuring cell: 四点法膜导电率测定Fourier’s law: 傅里叶定律Freezing point depression: 冰点抑制法Free-electron model: 自由电子模型Free volumes: 自由体积Frictional pressure drop: 摩擦压降Feedback loop: 反馈回路Fermi level: 费米能级Fixed stoichiometry condition: 固定化学计量比Flip-flop designs: 触发器设计Flow channels: 流道Flow field plates: 流场板Forced convection: 强迫对流Formation enthalpy: 生成焓Formic acid: 甲酸Forward activation barriers: 正向活化能Forward current density: 正向电流密度Forward flux: 正向通量Freezing/thawing: 冻结/融化Fuel crossover: 燃料穿透性Fuel processors: 燃料处理器Fuel reformers: 燃料重整器Fuel cell vehicles: 燃料电池汽车Fuel cell stacks: 燃料电池堆Fuel cell systems: 燃料电池系统Galvanostatic techniques: 恒流技术Gas-phase mole fraction of species i: i粒子气相摩尔分数GDL de-wetting and voltage loss: 气体扩散层脱湿及电压损失Green house gas emissions: 温室气体排放Gross heating value: 总热值Gibbs free energy: 吉布斯自由能Gadolinium-doped ceria: 钇掺杂的氧化铈Galvanic cell: 原电池(伽伐尼电池)Galvani potential: 伽伐尼电压Gas flow channels: 气体流道Gas diffusion layer: 气体扩散层Gasification: 气化Gasoline vehicle supply chain: 汽油车供应链Generalized mass-transfer driving force: 广义的传质驱动力Geometric design guidelines: 几何设计指南Global warming potential: 全球变暖潜力Governing equations: 控制方程Gravimetric energy density(specific energy): 重量能量密度(比能量)Gravimetric Power density(specific power): 重量功率密度(比功率)Gravimetric Ragone plots: 重量比功率-比能量曲线图Heat capacity: 热容Heat capacity effects:热容效应Heat and mass transfer: 传热传质Hydrogen reduction reaction: 氢还原反应Heat conduction: 热传导Heat pipe effect: 热管效应Heat radiation: 热辐射Heat spreaders cooling: 散热器冷却Heat transfer modes: 传热模式Heat engine: 热机Heat management: 热管理Heat release: 放热Heat exchangers: 热交换器Henry’s constant: 亨利常数Heat recovery efficiency: 热回收效率Helmholtz free energy: 亥姆霍兹自由能Heterpoly acid catalyst: 杂聚酸催化剂High current density region: 高电流密度区High-frequency intercept: 高频截距High-potential-energy initial state: 高势能初始状态High-temperature fuel cells: 高温燃料电池High-temperature oxidizing: 高温氧化Honda Home Energy Station: 本田家庭能源电站Hopping activation barrier: 跃迁活化能垒Hopping mechanisms: 跃迁机理Hopping model: 跃迁模型Hot reformate stream: 热重整气流Heuristic percolation theory: 启发式渗流理论High-frequency resistance: 高频阻抗Higher heating value: 高热值Hydrocarbon fuel: 碳氢燃料Hydrocarbon polymer: 碳氢高聚物Hydrogen-oxygen combustion reaction: 氢-氧燃烧反应Hydrogen-oxygen electrochemical reaction: 氢-氧电化学反应Hydrogen oxidation reaction: 氢气氧化反应Hypothetical condition: 假设条件Hydrogen utilization: 氢气利用率Humidification changes: 湿度变化Hydraulic permeation: 液压渗透Hydrogen and oxygen concentration contours: 氢气氧气浓度轮廓图Hydrophilic GDL: 亲水气体扩散层Hydrophobic GDL: 疏水气体扩散层Hydrostatic pressure: 静水压Hydrogen-hydrogen bond: 氢键能Hydrophobic treatment: 疏水处理Hydroscopic oxide: 吸湿氧化物Hydroxide compounds: 氢氧化物Hydroxy radicals: 氢氧自由基ICE(internal combustion engines):内燃机Ideal gas: 理想气体Impedance behavior: 阻抗行为Impedance response: 阻抗反应Incremental voltage loss: 增量式电压损失Inertial forces: 惯性力Infinite Warburg impedance element: 无限沃伯格阻抗元素Infinite Warburg behavior: 无限沃伯格行为Ink deposition: (催化剂)喷墨技术Inlet condition: 入口条件In situ electrochemical characterization techniques: 电化学原位表征技术Interdigitated flow: 交指型流动Interfacial potential: 界面电压Intermediate-strength bond: 中强化学键Internal fluid friction: 内流摩擦Internuclear separation: 核间距Intrinsic carriers: 內源载体Internal energy: 内能Intrinsic hopping rate: 内在跃迁速率Irreversible reaction process: 不可逆反应过程Isothermal reaction: 等温反应Ice dynamics and removal: 成冰动力学及除冰Ice formation: 结冰Ice melting: 冰融化Ice volume fraction: 冰体积分数Impedance model: 阻抗模型Ionic conduction: 离子传导Ionic conductors: 离子导体Ionic/electronic conductivities: 离子/电子导电率Ionic charge transport: 离子电荷传输Iterative process: 迭代过程Joules heat: 焦耳热Jump attempt frequency: 频率跃变Kinetic energy: 动能Kinetic Monte Carlo technique: 动力学蒙特卡洛技术Kinetic properties: 动力学性质KOH aqueous solution: 氢氧化钾水溶液Kinematic viscosity: 动力学粘度Knudsen diffusion: 努森扩散Lambert-Beer law: 朗伯-比尔定律Laminar flow: 层流Lanthanum gallate(LaGaO3): 酯酸镧Lanthanum Strontium Manganite(LSM):掺锶的亚锰酸镧Law of conservation of energy: 能量守恒定律Life cycle assessment: 生命周期评价Linear approximation: 线性逼近Linear voltage gradient: 线性电压梯度Linear Tafel fit: 线性塔菲尔拟合Liquid-gas transport models: 气-液传输模型Liquid-tin anode SOFC: 液态锡阳极SOFCLiquid water dynamics and removal: 液态水动力学及除水Liquid water transport model: 液态水传输模型Liquid saturation contours: 液态饱和廓线Lumped-system model: 总系统模型Logarithmic form: 对数形式Lone pair substitution: 孤对电子取代Low carbon fuels: 低碳燃料Low current densities: 低电流密度Low-potential-energy final state:低势能终态Low-temperature fuel cells: 低温燃料电池Lower heating value: 低热值Lumped-system model: 总系统模型Mass transfer: 传质Mass and momentum conservation: 质量和动量守恒Membrane hydration: 膜的湿化Microporous layers: 微孔层Macroscopic model prediction:宏观模型预测Mass and momentum conservation:质量与动量守恒Maxwell-Stefan flux equations: 麦克斯韦-斯特凡通量方程Molten carbonate fuel cells: 熔融碳酸盐燃料电池Membrane electrode assembly: 膜电极Membrane ionic conductivity: 膜离子导电率Membrane resistance profiles: 膜阻抗特征Methane: 甲烷Methanol: 甲醇Migration energy barrier: 迁移能垒Micro- , macro-transport: 微观,宏观传输Multiphase flow: 多相流Multiple-layered cathode catalyst layers: 多层阴极催化层Mass flow controllers: 质量流量控制器Matrix material: 基体材料Mechanical integrity: 机械优异性Membrane degradation: 膜降解Metal-air cells: 金属-空气电池Metal-based GDL materials: 金属基扩散层材料Metal hydride: 金属氢化物Metallic electron conductor: 金属电子导体Metal surfaces: 金属表面MEA fabrication: 膜电极制备Minimum temperature difference: 最小温差Mixed ion-conducting and electronically conducting: 混合离子-电子传导Molecular weight: 分子量Mobile fuel cell application: 手机燃料电池应用Modeling two-phase flow: 模拟两相流Monitoring system: 检测系统Molar concentration: 摩尔浓度Molar flow rate: 摩尔流速Molar flux: 摩尔通量Molar reaction quantities: 摩尔反应量Molar-weighted reactant: 摩尔加权反应Multicomponent gas diffusion: 多组分气体扩散Multiphase mixture formulation: 多相混合物配制Nafion:杜邦公司生产的质子交换膜,商品名,分117,115,112,212系列Nafion-based fuel cell system: 基于Nafion膜的燃料电池系统Natural desulfurized gas fuel: 天然脱硫气体燃料Natural gas fuel: 天然气燃料Natural greenhouse effect: 天然气温室效应Negative energy change: 负能量变化Nernst equation: 能斯特方程Nernstian concentration losses: 能斯特浓度损失Nernst voltage: 能斯特电压Net electrical efficiencies: 净电效率Net flux: 净通量Net reaction rate: 净反应速率Neutral water balance: 中性水平衡Net current extraction: 静电流输出Neutron radiography: 中子照相Net water transport coefficient: 净水传输系数Neutron radiography: 中子成相Newton’s law of cooling: 牛顿冷却定律Nickel-based electrodes: 镍基电极Nitrates: 硝酸盐Nitrogen flux: 氮气通量Nonplatinum ORR catalysts: 非铂氧气还原反应催化剂Nonuniformities: 非均一性Nonspontaneous reaction: 非自发反应Norminal diffusivity: 校正扩散Numerical analysis: 数值分析Nusselt number:努赛尔数Nyquist diagram/plot: 奈奎斯特图One-dimensional analysis: 一维分析Operational sweet spot: 操作优化点Ohmic loss: 欧姆损失Ohm’s law: 欧姆定律Ohmic voltage loss: 欧姆电压损失Ohmic activation loss: 欧姆活化损失Ohmic overvoltage: 欧姆过电位Ohmic resistance: 欧姆电阻Overall efficiency: 整体效率Oxygen/water concentration: 氧气/水浓度ORR(oxygen reduction reaction): 氧气还原反应Open circuit voltage: 开路电压Ordinary differential equation: 常微分方程Optical microscopy: 光学显微镜On-board fuel processor: 板载燃料处理器One-phase AC power: 单相交流电源Operating temperature: 操作温度Organic matter: 有机物质Orthorhombic tungsten bronze: 斜方晶钨青铜Outlet condition: 出口条件Oxidant species: 氧化剂粒子Oxide electrolytes: 氧化物电解质Oxide ions: 氧离子Oxygen-deficient perovskites: 缺氧型钙钛矿Oxygen-oxygen bonds: 氧-氧(共价)键Oxygen vacancies: 氧空位(穴)PEM fuel cell: 质子交换膜燃料电池Parasitic nonelectrochemical reactions: 寄生的非电化学反应Parasitic power: 寄生功率Partially humidified air: 部分加湿的空气Partial oxidation: 部分氧化PBI(polybenzimidazole): 聚苯并咪唑(一种高温质子交换膜)Phase change:相变Phase equilibrium: 相平衡Phase mobility: 相流动性Phase-change-based cooling: 基于相变的冷却Polymer electrolyte membrane fuel cell: 聚合物电解质膜燃料电池Pore-level heat transfer: 孔隙传热Pore-scale transport: 孔尺度传输Porous media: 多孔介质Power density: 功率密度Power-law conductivity model: 幂律特征的导电率模型Proton conductivity models: 质子导电模型Proton transport: 质子传输Phosphoric acid fuel cell: 磷酸燃料电池Palladium membrane: 钯膜Parallel flow: 平行流Power control unit: 动力控制单元PEEK(polyether ether ketone): 聚醚醚酮(一种低温质子交换膜)Perfectly smooth electrode-electrolyte interface: 完美的电极-电解质界面Perfluorinated polymers: 全氟聚合物(以Nafion为代表)Permeability: 渗透率Perovskite anode materials: 钙钛矿阳极材料Perovskite structure: 钙钛矿结构PTFE(Polytetrafluoroethylene): 聚四氟乙烯Phase-shifted current response: 基于相转移的电流响应Phosphoric acid doped PBI: 磷酸掺杂PBI膜Phosphotungstic acid: 磷钨酸Pinch point analysis: 夹点分析Planar connection designs: 面连接设计Planck’s constant: 普朗克常数Platinum-free catalysts: 非铂催化剂Poisoning effect: 中毒效应Polarization curve: 极化曲线Polymer: 高分子,聚合物Polymer chains: 高聚物链Power density: 功率密度Polymer ion conductors: 高聚物离子导体Pore size distributions: 孔径分布Porosity: 孔隙率Portable fuel cell: 可携带燃料电池Potassium hydroxide: 氢氧化钾Potential energy: 势能Pre-leaching process: 预浸工艺Pressure gauges: 压力表Pressure-swing absorption: 变压吸附Pressurized fuel cell system: 增压燃料电池系统Primary fuel reforming reactions: 主要燃料重整反应Proton conducting polymer: 质子导电聚合物Pt-based catalyst: 铂基催化剂Pulse-width voltage modulation: 脉宽电压调制方式Pyrochlore-type oxides: 焦绿石型氧化物Physical properties: 物理性质Planar structures: 面内结构Platinum electrodes: 铂电极Potentiostatic techniques: 恒压技术Proton transport: 质子传输Phase-change-based cooling: 基于相变的冷却Quantum mechanics: 量子力学Radiator: 散热器Reaction spontaneity: 反应自发性Reduction reaction: 还原反应Relative humidity:相对湿度Renewable fuel: 可再生燃料Reversible thermodynamic efficiency: 可逆热力学效率Reversible voltage: 可逆电压Reaction enthalpy: 反应焓Reverse current density: 反向电流密度Reynolds number: 雷诺数Radial frequency: 径向频率Reactants concentration: 反应物浓度Reaction rates: 反应速率Real fuel cell efficiency: 真实燃料电池效率Redox active dye molecules: 氧化还原活性染料分子Reforming process: 重整工艺Relaxation parameters: 松弛参数Resistors: 电阻器Reverse current density: 可逆电流密度Reverse reaction: 可逆反应Reversible thermodynamic efficiency: 可逆热力学效率Rotational diffusion transfer mechanisms: 旋转扩散传输机制Ruthenium: 钌Salt bridge: 盐桥Salt water battery: 盐水电池Scenario analysis: 方案分析Sealing method: 密封方法Solid state ionic conductor: 固态离子导体Spatially varying properties: 空间变化特性Species convection: 离子对流State of subfreezing water: 零下水状态Steady-state galvanostatic measurement: 静态恒流测试Steady-state properties:静态性质Stoichiometric number: 化学计量数Sulfonic group: 磺酸基Supply chain: 供应链Species transport equation: 粒子传输方程Stochastic material reconstruction: 随机材料重构Scaling and dimensionless groups: 缩放及无量纲组Schmidt number: 施密特数Second law of thermodynamics: 热力学第二定律Serpentine flow: 蛇形流Sherwood number: 舍伍德数Solid oxide fuel cells: 固体氧化物燃料电池Stanton number: 斯坦顿数Stefan-Boltzmann constant: 斯特凡-波尔兹曼常数Stefan-Boltzmann law: 斯特凡-波尔兹曼定律Samaria-doped ceria: 氧化钐掺杂的氧化铈Scanning electron microscopy: 扫描电子显微镜Sealant degradation: 密封胶降解Selective oxidation reactor: 选择性氧化反应器Semi-empirical expression: 半经验方程Shear force: 剪切力Shorting: 短路Silicotungstic acid: 硅钨酸Sintering: 烧结Sinusoidal current response:正弦电流响应Step-down converter: 降压转换器Step-up converter: 升压转换器Sulfonated polymer: 磺化聚合物Sulfonated hydrocarbon polymers: 磺化碳氢聚合物Symmetry condition: 对称条件Tafel equation: 塔菲尔方程Tafel slope: 塔菲尔斜率Thermal expansion coefficient: 热膨胀系数Thermal subsystem: 热系统Thermal conductivity: 热导率Thermodynamic voltage: 热力学电压Transport delay: 传输延迟Thermodynamics: 热力学Teflon: 聚四氟乙烯Temperature evolution and voltage loss: 温度变化与电压损失Transient phenomena: 瞬态现象Transversal membrane hydration: 横向膜加湿Transmission electron microscopy: 透射式电子显微镜Triple phase boundary: 三相界面Triple phase zones: 三相反应区Temperature-enthalpy diagram: 热-焓图Thermal balances: 热平衡Thermodynamics: 热力学Three-dimensional analysis: 三维分析Test conditions: 测试条件Turbulent flow: 湍流Two-fluid modeling:双流体模型Two-phase flow patterns: 两相流模式Vapor-phase diffusion: 气相扩散Vapor-liquid phase change: 气-液相变Variable-flow model: 变流模型Vehicular-diffusion mechanism: 汽车式扩散机制Volumetric condensation/evaporation rate: 体积冷凝/蒸发速率Volumetric storage capability: 容积储存能力Vacancies:空穴Vaporization latent heat: 蒸发潜热Viscous drag interaction: 粘性阻力作用Viscous forces: 粘性力Volatile organic compounds: 挥发性有机物Volumetric air flow rate: 体积空气流速Volumetric power density: 体积功率密度Void space: 空隙间隔Vulcan XC-72: 炭黑催化剂,商品名Water content: 含水量Water droplet dynamics: 水滴动力学Water holding capacity: 保水容量Water vapor saturation concentration: 水气饱和浓度Weber number: 韦伯数Wall condition: 壁面(边界)条件Water back diffusion: 水的反扩散Water gas shift reactors: 水气转换反应器X-ray diffraction(XRD): X射线衍射X-ray imaging: X射线成像Yttria-stabilized zirconia: 氧化钇稳定的氧化锆Zinc-air cell: 锌空电池Zirconia: 氧化锆Zirconium cations: 氧化锆阳离子Zr4+。
- 1、下载文档前请自行甄别文档内容的完整性,平台不提供额外的编辑、内容补充、找答案等附加服务。
- 2、"仅部分预览"的文档,不可在线预览部分如存在完整性等问题,可反馈申请退款(可完整预览的文档不适用该条件!)。
- 3、如文档侵犯您的权益,请联系客服反馈,我们会尽快为您处理(人工客服工作时间:9:00-18:30)。
RO P RO
RO OR RO P
R N R
PAr2
O P OR O
*L1
O R1 P N 2 R O
*L2
phosphine
(膦配体)
phosphonite
(亚膦酸酯)
phosphite
(亚磷酸酯)
phosphoamidite
(亚膦酸胺酯)
磷手性中心的手性双膦配体的 问题: 1) 制备困难; 2) 较高温度下消旋。
R Me
P
P
Me R t-Bu P H P t-Bu
BisP
磷手性中心的手性双膦配体的 优点: 1)手性三烷基膦配体中有位阻 大的较稳定,不易消旋。 2)与金属生成五员环的螯合配 合物。 3)手性中心邻近反应中心。
TangPhos
过渡金属与双齿配体的八面体络合物有两种构型:和,显示出手性。
采用有C2对称轴的手性配体可减少过渡态立体构型的数目, 而有利于立体控制; 两个萘环的高度扭曲的趋向是不对称诱导的决定性因素, 同时,二面角可调节; 结构刚性,旋转位垒高,不易外消旋。形成结构明确的只具 有sp2碳原子的七员螯合环。
O O
PPh2 PPh2
DIOP
O O
PPh2 PPh2
O O
PPh2 PPh2
(S,R,R,S)
使较柔软的DIOP更刚性,引入甲基……
(S,S,S,S)
R
+ COOR" [S,S)-(EtDuPhos)Rh] H2 OR'
R
COOR" OR'
在Rh-催化的芳烯胺的氢化反应中,
(S,R,R,S)-DIOP 给出优异的对映选择性,
若加入手性二胺,会有双不对称诱导的作用
(matched 和 mismatched)。
二.用于不对称氢化反应的手性配体
2.1.手性双膦配体
(1) 联芳环类配体
A) 联二萘类双膦配体
(R)-BINAP ) BINAP (S) BINAP (S)-BINAP PPh2 PPh2 PPh2 PPh2
八面体手性
(a) 二茂铁骨架
LA
LB
O Ph N O NH O
H2 Rh-LA (0.2mol%)
O Ph H O N NH H O
Vitamin H
COOMe
H
H2 Ru-LB
COOMe H
合成香料中间体 Angew, 2001, 40, 914 JACS, 1997, 6207
(R)-, up to 99% ee
H H OPPh2 OPPh2 BICOP
SpirOP (Chan & Jiang, 1997)
合成上的困难,促进结构设计加以改进
PAr2 PAr2
R
NHCOMe
SpirOP BICOP
> 99.9% ee 94% ee
(更刚性)
(Zhou,2003)(S)-SDP
Chan, Jiang, JACS, 1997, 119, 9570
PPh2 PPh2
PPh2 PPh2
(R)-BINAP )
(S) (S)-BINAP
Ar2 P Ru(OAc)2 P Ar2
COOH Ph NHAc
L*-Rh(I), H2 Ph
COOH NHAc
O N(CH3)2 O R OH
H2
OH N(CH3)2
对双键的不对称氢化反应很有效
JACS 1980, 102, 7932
O
O
而用BINAP做催化剂,该反应仅有84%ee
4
(4) 脂肪族双膦配体 (DIOP 和 DuPhos)
(a) 酒石酸衍生双膦配体 (DIOP)
(b) DuPhos---杜邦公司 (Burk) PennPhos具有更
大的刚性和立体位 阻。手性识别能力 更强。
PennPhos
R
R P P RR
DuPhos
P
CH2*CH(Me)(Et) CH2*CH(Me)(Et)
Me *P n-Pr 28% ee
Me *P i-Pr 28% ee OMe Me *P Cy (CAMP) 88% ee
1% ee
Me *P Cy 32% ee
OMe Me *P Ph (PAMP) 58% ee
筛选手性膦配体:
磷手性中心配体有很好的不对称诱导。
L Rh L
Cl L
Rh(I) 16e
H2, -L
Oxidative addition
L H Cl Rh L H
Rh(III) 16e
L H Cl -complex Rh Rh(III), 18e L H
三.不对称氢化反应的三类底物
1.C=C 双键的不对称催化氢化反应 2.羰基化合物的不对称氢化反应 3.亚胺的不对称氢化反应
第五章 不对称氢化和还原反应
一.历史的回顾 二.用于不对称氢化反应的 手性配体
1.手性双膦配体 2.手性单膦配体 3. 手性N,P N,P-和N,N N,N-配体
一.历史的回顾
a) 1960年代前,非均相催化的不对称氢化反应 b) 1965年, 发明了可溶催化剂的均相氢化反应:Rh(PPh3)3Cl, 1973年, Wilkinson获诺贝尔化学奖
O
O O
PPh2 PPh2
Optimal Ligand
C 3-TunePhos
(d) 联吡啶类双膦配体—P-PHOS
(c) H8-BINAP
R1 MeO MeO
R2
R3 PAr2 PAr2
OMe N PAr2 PAr2 N MeO MeO N OMe P-PHOS, >99% ee (Chan, 2002) N PAr2 PAr2
Ph
螺环结构的优点: 与联二萘具有相同的C2对称性,但具有 相对高的刚性和优良的不对称性。
Xyl-SDP S/C 100,000, conv. 98%, ee 98%
Zhou, JACS, 2003, 125, 4404
(3) 平面手性双膦配体
Me
(b) PHANEPhos-Rh催化剂
PPh2 P(t-Bu)2 Fe Me Fe PCy2 P(p-C6H5CF3)2
*P
R1 R3 R2
* R1
P R3 R2
*
*P
R1 R3 R2
“双齿”配体的优越性。 取代基的适当位置有第二个配位点,对映选择性有很大提高。
d) 随后Kagan发明了双齿膦配体—DIOP
(香草醛)
DIOP-Rh(I)配合物催化-(酰氨基)丙烯酸(酯)的不对称氢 化反应 e.e.值达到80%以上。 PAMP二聚给出DIPAMP。易制备, 空气中生成稳定的晶体
More active
(S )
H2 Ar2 Ph N (S) P H Ru P Cl N (S) Ar2 H2 Ph
less active
R2 O R1 R3 R4
H2 R1
R2 OH R4 R3
特点: 适于非官能化的酮的氢化反应
对,-不饱和酮有很高的化学选择性和立体选择性。 只氢化羰基而保留双键,ee值可高达100%。 催化剂中的苯基换成大位阻基团给出更好的选择性。
95% ee
手性位于碳骨架上能与金属形成螯合配合物,发挥手性诱 导作用。---Kagan发明的意义
1
e) 1980年Noyori报道了BINAP-Rh(I)配合物催化的 不对称氢化反应
f) 1986年,使用BINAP-Ru(II)-(OAc)2又是一个重大的突破 (I)以Ru(II)/BINAP配合物作催化剂可以扩展到官能化的酮
2
B) 联二苯双膦配体 联二芳环的二面角(dihedral angle)对对映选择性有很大的影响!! (X. Zhang, “New Chiral Phosphorus Ligands for Enantioselective Hydrogenation”, Chem. Rev. 2003, 103, 3029)
PPh2 PPh2
R1
R2
R3
吡啶基团的氮原子也能参加配位,使中心金属的配位达到饱和,降低 了活性。周围引入一定体积的取代基后可防止氮原 子与催化剂中金属
BINAP经还原有一个苯环成脂肪环,改变了二面角, 在 Ru- 催 化 的 不 饱 和 羧 酸 的 氢 化 应 中 H8-BINAP 比 BINAP表现出更好的对映选择性。
H- transfer
RhClL3 + CH3CH3
四. 除氢化反应外的还原体系
+L Reductive elimination
L H Cl -complex Rh(III), 16e Rh L CH2CH3
c) 1968年,Knowles以手性膦配体代替三苯基膦 以-(酰氨基)丙烯酸(酯)的不对称氢化反应为模型反应
原子的配位,增加催化剂的活性。 Ar改用大位阻基团,选择性更好 适用于各种不同的底物 催化剂对空气和水不敏感,甚至可用水处理。可大规模制备
Chan, A. S. C.,JACS,2000,11513
3
(2) 螺环双膦配体
* R * Spiro[4,4]-nonane Ph2P * O O * *
O (CH2)n O
n = 1~6
PPh2 PPh2
Dihedral Angle (°) Substrate
O N O
Compare E.e.(%)
91 3 90.3 91.3 90 3 98.5 98 5 95.1 95 1 95.3 95 3 90.7 90 7
96 1 96.1
94 3 94.3
R=P (?)