钠离子电池电解液文献
钠电池电解液

钠电池电解液
钠电池电解液是一种应用广泛的电池类型,它在各种电子设备和能源存储系统中发挥着重要作用。
本文将就钠电池电解液的组成、特性和应用进行介绍,希望能够让读者对这一领域有更深入的了解。
钠电池电解液通常由溶剂、钠盐和添加剂组成。
溶剂可以是有机溶剂或水系溶剂,主要用于溶解钠盐和其他添加剂,以提供良好的离子传导性能。
钠盐作为电解质,能够在电池的正负极之间传递钠离子,从而实现电荷和放电过程。
添加剂则用于调节电解液的粘度、稳定性和电化学性能,以确保电池的高效运行。
钠电池电解液具有许多优点,例如较高的能量密度、较长的循环寿命和较低的成本。
与传统的锂离子电池相比,钠电池电解液更加环保,因为钠是地球上丰富的资源,而且价格相对较低。
此外,钠电池电解液的循环寿命较长,能够经受更多的充放电循环,同时也具备更好的安全性能,减少了电池发生短路或爆炸的风险。
钠电池电解液在能源存储系统中有着广泛的应用。
它可以用于储能设备、电动汽车、太阳能和风能发电系统等领域,为人们的生活和工作提供持续稳定的能源供应。
特别是在可再生能源领域,钠电池电解液的应用将有助于解决能源存储和供应不稳定的问题,推动清洁能源的发展和利用。
总的来说,钠电池电解液作为一种重要的电池材料,在能源领域具
有巨大的潜力和发展空间。
随着科技的不断进步和创新,相信钠电池电解液将会在未来发挥更加重要的作用,为人类社会的可持续发展和繁荣做出贡献。
希望本文的介绍能够帮助读者更好地了解钠电池电解液的特性和应用,促进该领域的研究和发展。
钠离子电池离子液体电解液的配制与性能表征-2018
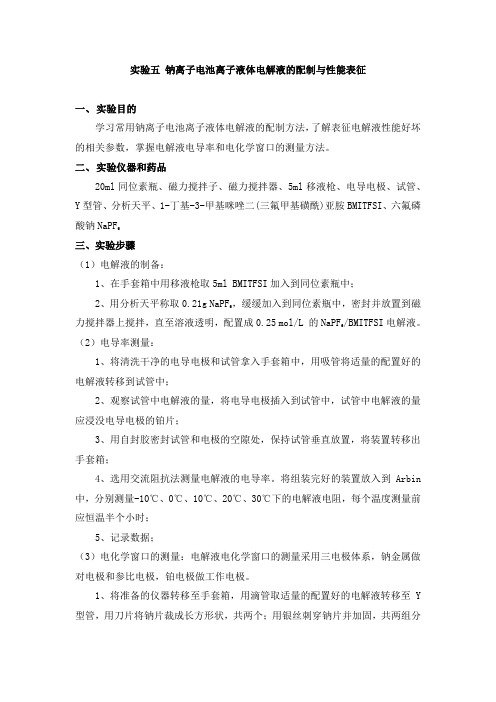
实验五钠离子电池离子液体电解液的配制与性能表征一、实验目的学习常用钠离子电池离子液体电解液的配制方法,了解表征电解液性能好坏的相关参数,掌握电解液电导率和电化学窗口的测量方法。
二、实验仪器和药品20ml同位素瓶、磁力搅拌子、磁力搅拌器、5ml移液枪、电导电极、试管、Y型管、分析天平、1-丁基-3-甲基咪唑二(三氟甲基磺酰)亚胺BMITFSI、六氟磷酸钠NaPF6三、实验步骤(1)电解液的制备:1、在手套箱中用移液枪取5ml BMITFSI加入到同位素瓶中;,缓缓加入到同位素瓶中,密封并放置到磁2、用分析天平称取0.21g NaPF6/BMITFSI电解液。
力搅拌器上搅拌,直至溶液透明,配置成0.25 mol/L 的NaPF6(2)电导率测量:1、将清洗干净的电导电极和试管拿入手套箱中,用吸管将适量的配置好的电解液转移到试管中;2、观察试管中电解液的量,将电导电极插入到试管中,试管中电解液的量应浸没电导电极的铂片;3、用自封胶密封试管和电极的空隙处,保持试管垂直放置,将装置转移出手套箱;4、选用交流阻抗法测量电解液的电导率。
将组装完好的装置放入到Arbin 中,分别测量-10℃、0℃、10℃、20℃、30℃下的电解液电阻,每个温度测量前应恒温半个小时;5、记录数据;(3)电化学窗口的测量:电解液电化学窗口的测量采用三电极体系,钠金属做对电极和参比电极,铂电极做工作电极。
1、将准备的仪器转移至手套箱,用滴管取适量的配置好的电解液转移至Y 型管,用刀片将钠片裁成长方形状,共两个;用银丝刺穿钠片并加固,共两组分别为参比电极和工作电极;2、将铂电极和钠金属参比电极共同放入Y型管一侧,钠金属对电极放入Y 型管另一侧,调整钠片位置使得电解液能够淹没钠片,固定电极并转移出手套箱;3、电化学窗口的测量:采用循环伏安法测量电解液的电化学窗口,测量电势范围-1-6V,扫描周期为3。
四、数据分析(1)电导率计算:对所配置电解液采用交流阻抗法进行变温电导率测试。
水系钠离子电池正极材料及电解液研究进展
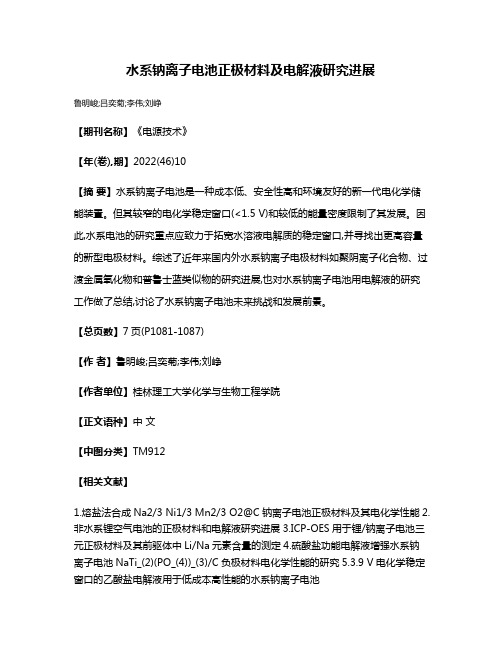
水系钠离子电池正极材料及电解液研究进展
鲁明峻;吕奕菊;李伟;刘峥
【期刊名称】《电源技术》
【年(卷),期】2022(46)10
【摘要】水系钠离子电池是一种成本低、安全性高和环境友好的新一代电化学储能装置。
但其较窄的电化学稳定窗口(<1.5 V)和较低的能量密度限制了其发展。
因此,水系电池的研究重点应致力于拓宽水溶液电解质的稳定窗口,并寻找出更高容量的新型电极材料。
综述了近年来国内外水系钠离子电极材料如聚阴离子化合物、过渡金属氧化物和普鲁士蓝类似物的研究进展,也对水系钠离子电池用电解液的研究工作做了总结,讨论了水系钠离子电池未来挑战和发展前景。
【总页数】7页(P1081-1087)
【作者】鲁明峻;吕奕菊;李伟;刘峥
【作者单位】桂林理工大学化学与生物工程学院
【正文语种】中文
【中图分类】TM912
【相关文献】
1.熔盐法合成Na2/3 Ni1/3 Mn2/3 O2@C钠离子电池正极材料及其电化学性能
2.非水系锂空气电池的正极材料和电解液研究进展
3.ICP-OES用于锂/钠离子电池三元正极材料及其前驱体中Li/Na元素含量的测定
4.硫酸盐功能电解液增强水系钠离子电池NaTi_(2)(PO_(4))_(3)/C负极材料电化学性能的研究
5.3.9 V电化学稳定窗口的乙酸盐电解液用于低成本高性能的水系钠离子电池
因版权原因,仅展示原文概要,查看原文内容请购买。
钠离子电池电解质的研究进展
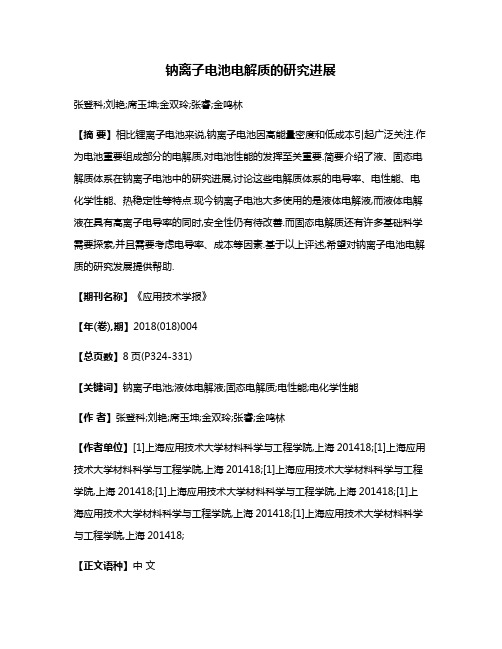
钠离子电池电解质的研究进展张登科;刘艳;席玉坤;金双玲;张睿;金鸣林【摘要】相比锂离子电池来说,钠离子电池因高能量密度和低成本引起广泛关注.作为电池重要组成部分的电解质,对电池性能的发挥至关重要.简要介绍了液、固态电解质体系在钠离子电池中的研究进展,讨论这些电解质体系的电导率、电性能、电化学性能、热稳定性等特点.现今钠离子电池大多使用的是液体电解液,而液体电解液在具有高离子电导率的同时,安全性仍有待改善.而固态电解质还有许多基础科学需要探索,并且需要考虑电导率、成本等因素.基于以上评述,希望对钠离子电池电解质的研究发展提供帮助.【期刊名称】《应用技术学报》【年(卷),期】2018(018)004【总页数】8页(P324-331)【关键词】钠离子电池;液体电解液;固态电解质;电性能;电化学性能【作者】张登科;刘艳;席玉坤;金双玲;张睿;金鸣林【作者单位】[1]上海应用技术大学材料科学与工程学院,上海201418;[1]上海应用技术大学材料科学与工程学院,上海201418;[1]上海应用技术大学材料科学与工程学院,上海201418;[1]上海应用技术大学材料科学与工程学院,上海201418;[1]上海应用技术大学材料科学与工程学院,上海201418;[1]上海应用技术大学材料科学与工程学院,上海201418;【正文语种】中文【中图分类】TM911.3随着人类社会不断发展,化石能源的消耗不断增加,能源危机、环境污染等问题越发严重。
近年来,随着潮汐能、太阳能等可再生能源的不断发展,作为影响其发展的关键技术,储能系统越来越受到人们的关注。
其中,二次电池以其效率高、使用方便等优点成为最具潜力的储能方式。
已经趋于商业化的二次电池有铅酸电池、锂电池、钠电池等,锂电池因能量密度高、自放电小、安全无污染等优势已经在通信电子产品领域得到大规模应用。
然而大规模工业储能需要储能器件满足2个基本要求:一是安全性高,没有安全就没有储能的未来;二是成本低,任何技术的发展必须考虑成本问题。
国外钠离子电池体系电解液离子电导率
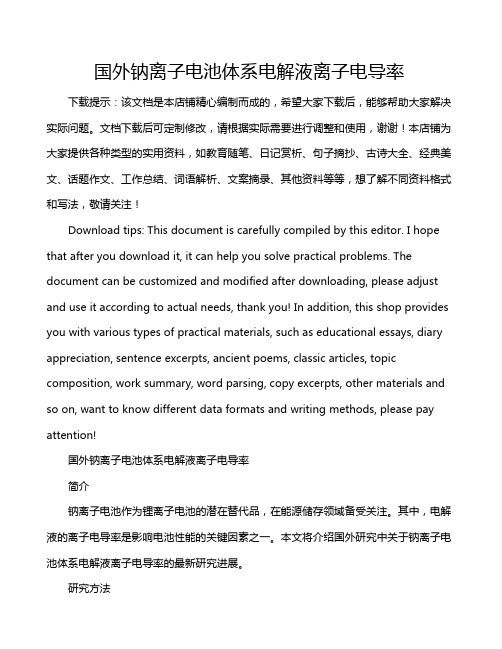
国外钠离子电池体系电解液离子电导率下载提示:该文档是本店铺精心编制而成的,希望大家下载后,能够帮助大家解决实际问题。
文档下载后可定制修改,请根据实际需要进行调整和使用,谢谢!本店铺为大家提供各种类型的实用资料,如教育随笔、日记赏析、句子摘抄、古诗大全、经典美文、话题作文、工作总结、词语解析、文案摘录、其他资料等等,想了解不同资料格式和写法,敬请关注!Download tips: This document is carefully compiled by this editor. I hope that after you download it, it can help you solve practical problems. The document can be customized and modified after downloading, please adjust and use it according to actual needs, thank you! In addition, this shop provides you with various types of practical materials, such as educational essays, diary appreciation, sentence excerpts, ancient poems, classic articles, topic composition, work summary, word parsing, copy excerpts, other materials and so on, want to know different data formats and writing methods, please pay attention!国外钠离子电池体系电解液离子电导率简介钠离子电池作为锂离子电池的潜在替代品,在能源储存领域备受关注。
钠离子电池研究进展

钠离子电池研究进展一、本文概述随着全球能源需求的持续增长,以及对环境保护和可持续发展的日益关注,高效、环保、可再生的能源存储技术已成为当前研究的热点。
其中,钠离子电池作为一种新型的二次电池,凭借其原料丰富、成本低廉、环境友好等优势,近年来受到了广泛的关注。
本文旨在综述钠离子电池的研究进展,包括其基本原理、材料体系、制造工艺以及应用前景等方面的内容,以期为钠离子电池的进一步研究和应用提供参考和借鉴。
通过深入了解钠离子电池的研究现状和发展趋势,我们有望为未来的能源存储技术找到更加环保、经济、高效的解决方案。
二、钠离子电池的基本原理与结构钠离子电池(Sodium-ion Batteries,SIBs)是一种新型的可充电电池技术,其基本原理与已广泛应用的锂离子电池(LIBs)相似,主要区别在于阳离子从锂变为钠。
钠离子电池的核心组成部分包括正极、负极、电解质以及隔膜。
正极材料:正极是钠离子电池的重要组成部分,其性能直接影响电池的能量密度和循环寿命。
目前,研究者们已经开发出多种适用于钠离子电池的正极材料,如层状氧化物(如NaCoO₂、NaMnO₂等)、聚阴离子型化合物(如NaFePO₄、Na₃V₂(PO₄)₃等)以及普鲁士蓝类似物等。
这些材料具有高的钠离子存储容量和良好的结构稳定性,使得钠离子电池具有较高的能量密度和循环稳定性。
负极材料:负极材料在钠离子电池中同样扮演着关键角色。
目前,碳基材料(如硬碳、石墨等)是最常用的钠离子电池负极材料,它们具有较高的比容量和良好的循环性能。
研究者们还在探索其他新型负极材料,如金属氧化物、硫化物和合金等,以进一步提高钠离子电池的性能。
电解质:电解质是钠离子电池中连接正负极的关键部分,负责离子的传输。
常用的电解质包括有机液体电解质、无机固体电解质和聚合物电解质等。
这些电解质需要具有良好的离子导电性、化学稳定性和机械强度,以确保电池的安全性和性能。
隔膜:隔膜位于正负极之间,用于防止电池内部短路。
钠离子电池醚类电解液分解
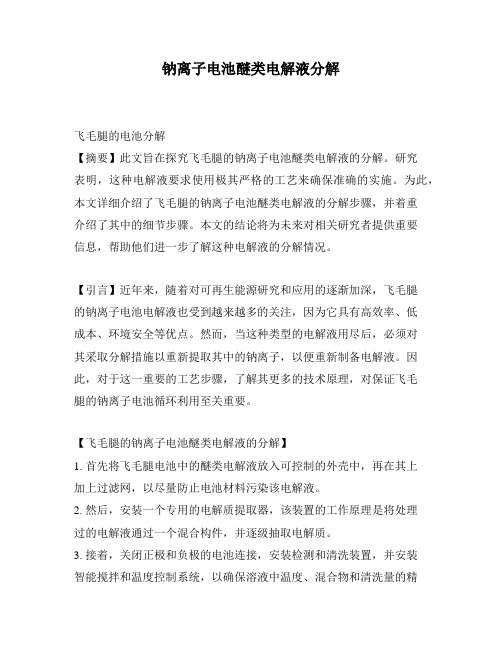
钠离子电池醚类电解液分解飞毛腿的电池分解【摘要】此文旨在探究飞毛腿的钠离子电池醚类电解液的分解。
研究表明,这种电解液要求使用极其严格的工艺来确保准确的实施。
为此,本文详细介绍了飞毛腿的钠离子电池醚类电解液的分解步骤,并着重介绍了其中的细节步骤。
本文的结论将为未来对相关研究者提供重要信息,帮助他们进一步了解这种电解液的分解情况。
【引言】近年来,随着对可再生能源研究和应用的逐渐加深,飞毛腿的钠离子电池电解液也受到越来越多的关注,因为它具有高效率、低成本、环境安全等优点。
然而,当这种类型的电解液用尽后,必须对其采取分解措施以重新提取其中的钠离子,以便重新制备电解液。
因此,对于这一重要的工艺步骤,了解其更多的技术原理,对保证飞毛腿的钠离子电池循环利用至关重要。
【飞毛腿的钠离子电池醚类电解液的分解】1. 首先将飞毛腿电池中的醚类电解液放入可控制的外壳中,再在其上加上过滤网,以尽量防止电池材料污染该电解液。
2. 然后,安装一个专用的电解质提取器,该装置的工作原理是将处理过的电解液通过一个混合构件,并逐级抽取电解质。
3. 接着,关闭正极和负极的电池连接,安装检测和清洗装置,并安装智能搅拌和温度控制系统,以确保溶液中温度、混合物和清洗量的精确控制。
4. 取出处理后的醚类电解液,经过滤和精馏,可以得到清洁、还原的电解液,可以重新加以使用。
【结论】以上就是飞毛腿的钠离子电池醚类电解液的分解的步骤,其过程严格考虑到每一个步骤的细节,也尤为重要。
此外,上述分解步骤还需借助相关的技术设备,对电池能量存储材料及应用环境要求非常苛刻。
因此,进行飞毛腿钠离子电池醚类电解液分解时,应按照上述步骤准确实施,严格控制过程中的温度,混合物浓度等参数,以保证正确的结果。
Na_(3)V_(2)(PO_(4))_(2)O_(2)F的合成及其在钠离子电池中的应用
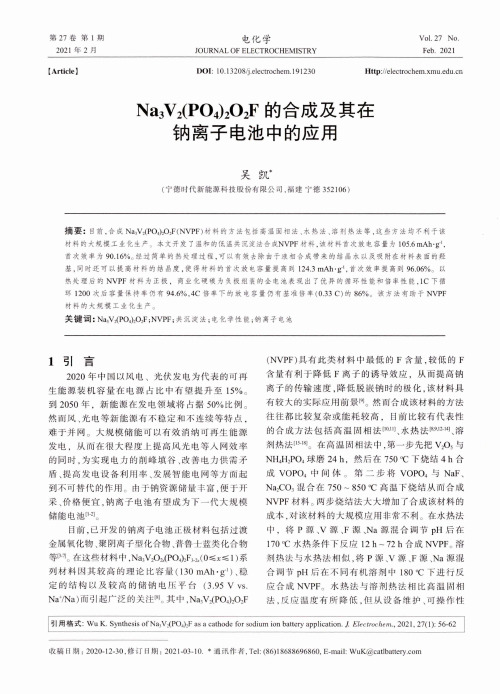
第27卷第1期电化学 VoL 27 No. 2021 年 2 月JOURNAL OF ELECTROCHEMISTRY Feb. 2021[Article]DOI: 10.13208/j.electrochem. 191230Na3V2(P04)202F的合成及其在钠离子电池中的应用吴凯*(宁德时代新能源科技股份有限公司,福建宁德352106)摘要:目前,合成NaWJPOAOWNVPF)材料的方法包括高温固相法、水热法、溶剂热法等,这些方法均不利于该 材料的大规模工业化生产。
本文开发了温和的低温共沉淀法合成N V PF材料,该材料首次放电容量为lOSAmAlvg-1, 首次效率为90.16%。
经过简单的热处理过程,可以有效去除由于液相合成带来的结晶水以及吸附在材料表面的羟 基,同时还可以提高材料的结晶度,使得材料的首次放电容量提高到124.3 m A h*g'首次效率提高到96.06%。
以热处理后的N V PF材料为正极,商业化硬碳为负极组装的全电池表现出了优异的循环性能和倍率性能,1C下循 环1200次后容量保持率仍有94.6%,4C倍率下的放电容量仍有基准倍率(0.33 C)的86%。
该方法有助于NVPF 材料的大规模工业化生产。
关键词:Na3V2(P04)202F; NVPF;共沉淀法;电化学性能;钠离子电池1引言2020年中国以风电、光伏发电为代表的可再生能源装机容量在电源占比中有望提升至15%。
到2050年,新能源在发电领域将占据50%比例。
然而风、光电等新能源有不稳定和不连续等特点,难于并网。
大规模储能可以有效消纳可再生能源发电,从而在很大程度上提高风光电等人网效率的同时,为实现电力的削峰填谷、改善电力供需矛盾、提高发电设备利用率、发展智能电网等方面起到不可替代的作用。
由于钠资源储量丰富,便于开 采、价格便宜,钠离子电池有望成为下一代大规模储能电池[“2]。
目前,已开发的钠离子电池正极材料包括过渡 金属氧化物、聚阴离子型化合物、普鲁士蓝类化合物 等叫。
钠离子电池用电解质钠盐的研究进展

Research progress in sodium salts electrolyte for sodium ion battery
LU Hong-li,YANG Li,WANG Jie,LI Shi-you
钠盐是 Na 的主要提供者, 不但影响电池的功率和循环性
+
能,还会影响容量和安全性。 钠盐需要满足以下要求:溶解
六氟磷酸钠( NaPF6 ) 、高氯酸钠( NaClO4 ) 和双三氟甲烷磺酰
性能钠离子电池的需求,如 NaClO4 毒性高、NaTFSI 对铝箔有
腐蚀性、NaPF6 易分解和水解等 [4] 。 要提高钠离子电池的性
and other electrolyte sodium salts were summarized. The structural characteristics of the electrolyte sodium salt were analyzed. The
mechanisms for the improving performance of sodium ion battery with salts due to the unique structure were illuminated. The research
( 兰州理工大学石油化工学院,甘肃 兰州 730050 )ቤተ መጻሕፍቲ ባይዱ
摘要:综述近年来含氰基类钠盐、酰胺类钠盐和氟类咪唑衍生物钠盐等电解质钠盐在钠离子电池中的研究应用。 分析这些
电解质钠盐的结构特征,阐述钠盐因独特结构优势提升电池性能的机理,展望钠离子电池电解质中钠盐的发展趋势。
钠离子电池新型电解液的研究

钠离子电池新型电解液的研究钠离子电池是一种适用于储能领域的电化学储能装置。
与锂离子电池相比,它具有更高的容量、更低的成本和更广泛的资源来源。
目前,钠离子电池的电解液采用有机溶剂,在温度和安全性方面还存在一些问题。
因此,开发一种高性能、低成本、绿色环保的新型电解液对于钠离子电池的实际应用至关重要。
一、有机溶剂电解液的问题目前,大多数钠离子电池采用有机溶剂作为电解液。
这些有机溶剂对于钠离子电池的电化学性能有很大的影响。
虽然它们具有较高的电化学稳定性,但同时它们也存在很多问题。
首先,它们的熔点低,易于引燃。
其次,它们的挥发性很大,易导致电池内部压力上升,进而导致电池的爆炸。
此外,它们还有可能导致电池内部产生副反应,进而降低电池的循环寿命。
因此,需要开发一种新型的电解液,以解决以上问题。
二、新型电解液的研究方向目前,新型电解液的研发方向主要集中在以下几个方面:1. 离子液体电解液离子液体是指在室温下能保持离子化状态的液体。
相较于溶解在有机溶剂中的电解质,离子液体更加稳定,且可重复使用。
因此,离子液体电解液成为了钠离子电池研究的一个热点。
然而,离子液体的成本较高,且目前存在的离子液体对钠离子的传输率较低,因此需要进一步优化。
2. 水性电解液水性电解液是指以水为基准的电解液。
相较于有机溶剂电解液,水性电解液具有更低的成本、更广泛的资源来源和更好的环境友好性。
不过,水性电解液也有很多需要解决的问题。
首先,水分解电压较高,会导致电池的额定电压下降;其次,水会引起金属钠电极的氧化和腐蚀,进而影响电池的循环寿命;再者,水性电解液的电导率相对较低,会降低电池的输出功率。
因此,需要优化水性电解液的电导率和稳定性。
3. 聚合物电解液聚合物电解液是指将聚合物作为载体的电解液。
相较于传统的有机溶剂电解液,聚合物电解液具有更高的电化学稳定性和更低的挥发性。
然而,聚合物电解液的电导率较低,需要进一步提高。
三、新型电解液的发展趋势未来,新型电解液的研究方向将持续扩展,不过优化传统的有机溶剂电解液仍将是很重要的一个方向。
钠离子电池电解液体系及其性能优化
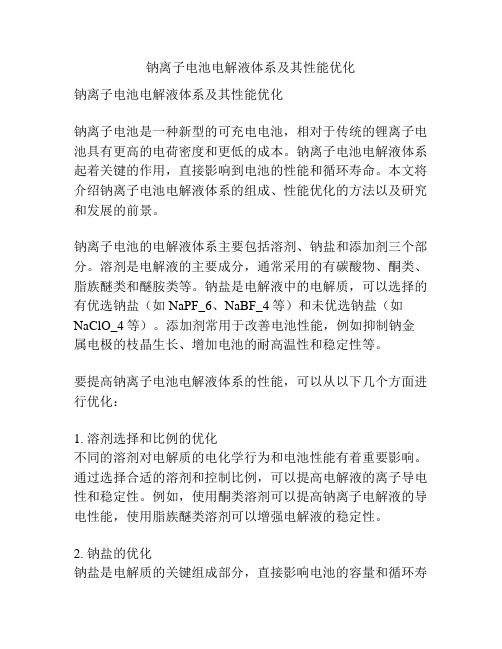
钠离子电池电解液体系及其性能优化钠离子电池电解液体系及其性能优化钠离子电池是一种新型的可充电电池,相对于传统的锂离子电池具有更高的电荷密度和更低的成本。
钠离子电池电解液体系起着关键的作用,直接影响到电池的性能和循环寿命。
本文将介绍钠离子电池电解液体系的组成、性能优化的方法以及研究和发展的前景。
钠离子电池的电解液体系主要包括溶剂、钠盐和添加剂三个部分。
溶剂是电解液的主要成分,通常采用的有碳酸物、酮类、脂族醚类和醚胺类等。
钠盐是电解液中的电解质,可以选择的有优选钠盐(如NaPF_6、NaBF_4等)和未优选钠盐(如NaClO_4等)。
添加剂常用于改善电池性能,例如抑制钠金属电极的枝晶生长、增加电池的耐高温性和稳定性等。
要提高钠离子电池电解液体系的性能,可以从以下几个方面进行优化:1. 溶剂选择和比例的优化不同的溶剂对电解质的电化学行为和电池性能有着重要影响。
通过选择合适的溶剂和控制比例,可以提高电解液的离子导电性和稳定性。
例如,使用酮类溶剂可以提高钠离子电解液的导电性能,使用脂族醚类溶剂可以增强电解液的稳定性。
2. 钠盐的优化钠盐是电解质的关键组成部分,直接影响电池的容量和循环寿命。
优选钠盐具有较高的溶解度和稳定性,可以提高电解液的离子导电性和充放电效率。
此外,钠盐的浓度也会影响电池的性能,适当的浓度可以提高电池的能量密度。
3. 添加剂的选择和比例的优化添加剂在钠离子电池电解液中起着重要的作用,可以改善电池的循环寿命和安全性能。
例如,添加锂盐可以抑制钠金属电极的枝晶生长,减少钠离子电池的枝晶短路问题。
添加抑制剂可以抑制氧化还原反应的副反应,提高电池的循环寿命和高温稳定性。
添加剂的选择和比例的优化需要综合考虑电池的具体条件和应用需求。
钠离子电池电解液体系的研究和发展还面临一些挑战,例如钠金属电极的界面问题、电解液的稳定性和循环寿命等。
目前的研究主要集中在电解液的化学成分和结构的优化、界面层的调控和构筑、电解液的固态化等方面。
钠离子电池用有机电解液标准_概述说明以及解释
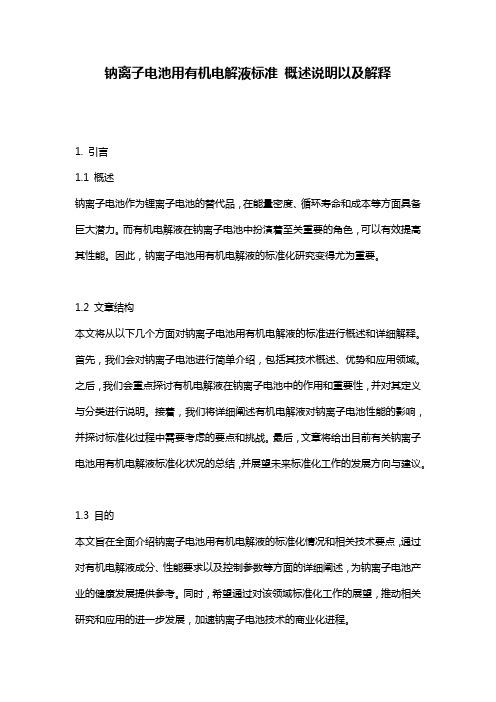
钠离子电池用有机电解液标准概述说明以及解释1. 引言1.1 概述钠离子电池作为锂离子电池的替代品,在能量密度、循环寿命和成本等方面具备巨大潜力。
而有机电解液在钠离子电池中扮演着至关重要的角色,可以有效提高其性能。
因此,钠离子电池用有机电解液的标准化研究变得尤为重要。
1.2 文章结构本文将从以下几个方面对钠离子电池用有机电解液的标准进行概述和详细解释。
首先,我们会对钠离子电池进行简单介绍,包括其技术概述、优势和应用领域。
之后,我们会重点探讨有机电解液在钠离子电池中的作用和重要性,并对其定义与分类进行说明。
接着,我们将详细阐述有机电解液对钠离子电池性能的影响,并探讨标准化过程中需要考虑的要点和挑战。
最后,文章将给出目前有关钠离子电池用有机电解液标准化状况的总结,并展望未来标准化工作的发展方向与建议。
1.3 目的本文旨在全面介绍钠离子电池用有机电解液的标准化情况和相关技术要点,通过对有机电解液成分、性能要求以及控制参数等方面的详细阐述,为钠离子电池产业的健康发展提供参考。
同时,希望通过对该领域标准化工作的展望,推动相关研究和应用的进一步发展,加速钠离子电池技术的商业化进程。
2. 钠离子电池简介:2.1 概述钠离子电池技术钠离子电池是一种新型的二次电池技术,类似于常见的锂离子电池。
它通过在正负极之间运输和储存钠离子来实现能量的存储和释放。
与传统的锂离子电池相比,钠离子电池具有更高的容量和更低的成本。
这使得它成为了可替代锂离子电池的一种潜在选择。
2.2 钠离子电池的优势和应用领域钠离子电池相较于其他二次电池技术具有几个重要优势。
首先,钠是地球上丰富而廉价的资源,远比锂更易获取。
其次,由于钠离子在水中运动速度较快,钠离子电池通常具有更高的充放电速率。
此外,相较于锂离子电池需要使用过氧化物、稀土等稀缺材料制备正负极材料,钠在正负极材料中广泛应用可以降低成本。
目前,钠除了用于大规模能源存储的领域外,还在电动汽车、可再生能源系统和便携式设备等应用中显示了巨大的潜力。
钠离子电池电解液 技术
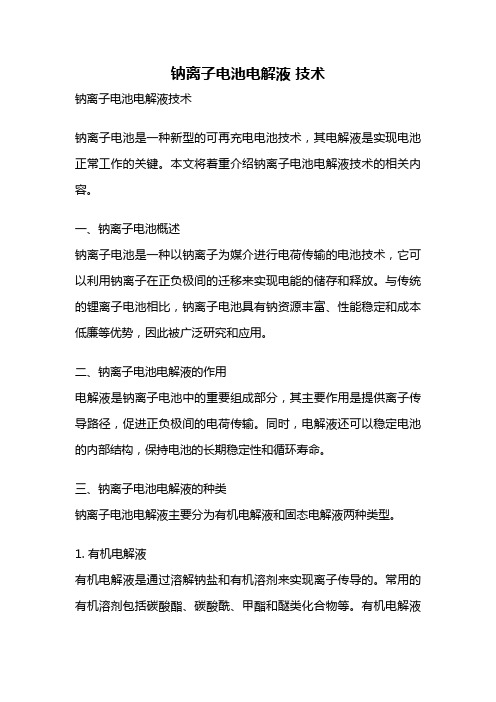
钠离子电池电解液技术钠离子电池电解液技术钠离子电池是一种新型的可再充电电池技术,其电解液是实现电池正常工作的关键。
本文将着重介绍钠离子电池电解液技术的相关内容。
一、钠离子电池概述钠离子电池是一种以钠离子为媒介进行电荷传输的电池技术,它可以利用钠离子在正负极间的迁移来实现电能的储存和释放。
与传统的锂离子电池相比,钠离子电池具有钠资源丰富、性能稳定和成本低廉等优势,因此被广泛研究和应用。
二、钠离子电池电解液的作用电解液是钠离子电池中的重要组成部分,其主要作用是提供离子传导路径,促进正负极间的电荷传输。
同时,电解液还可以稳定电池的内部结构,保持电池的长期稳定性和循环寿命。
三、钠离子电池电解液的种类钠离子电池电解液主要分为有机电解液和固态电解液两种类型。
1. 有机电解液有机电解液是通过溶解钠盐和有机溶剂来实现离子传导的。
常用的有机溶剂包括碳酸酯、碳酸酰、甲酯和醚类化合物等。
有机电解液具有导电性好、离子传输速度快等特点,但在高温下易挥发和燃烧,存在一定的安全隐患。
2. 固态电解液固态电解液是将钠盐固态化后与固态离子导体混合形成的。
固态电解液具有热稳定性好、安全性高等优点,但由于固态电解液的离子传导性较差,目前还存在着较大的改进空间。
四、钠离子电池电解液技术的研究进展钠离子电池电解液技术的研究主要集中在提高电解液的离子传导性、提高电池的能量密度和循环寿命等方面。
1. 寻找更好的离子导体研究人员正在探索新型的离子导体材料,以提高电解液的离子传导性。
例如,石墨烯、钙钛矿材料等具有良好导电性和离子传导性的材料被广泛应用于钠离子电池电解液中。
2. 优化电解液组分研究人员还尝试优化电解液的组分,以提高电池的能量密度和循环寿命。
例如,添加一定的添加剂可以改善电解液的稳定性和界面反应,减少电池的损耗。
3. 开发高性能固态电解液固态电解液因其优异的安全性和热稳定性备受关注,研究人员正在努力开发高性能的固态电解液。
例如,采用导电聚合物和陶瓷材料作为固态电解液的基质,可以提高电池的离子传导性。
钠离子电池技术的研究与应用

钠离子电池技术的研究与应用摘要钠离子电池作为一种新型的储能技术,具有较高的能量密度和较低的成本,因此受到了广泛关注。
本文综述了钠离子电池技术的研究进展和应用前景,包括钠离子电池的工作原理、正负极材料的选择、电解液的优化、电池性能的提升等方面。
同时,我们还讨论了钠离子电池在可再生能源储能、电动车辆、大规模能源存储等领域的应用前景,并提出了当前研究中存在的挑战和未来发展方向。
1. 引言随着全球经济的快速发展和环境问题的日益严重,储能技术在可再生能源利用和电动交通领域的重要性日益凸显。
钠离子电池作为一种新兴的储能技术,具有能量密度高、资源丰富和成本低等优势,在近年来得到了广泛的研究和关注。
2. 钠离子电池的工作原理钠离子电池与传统的锂离子电池类似,都是基于电化学反应来进行能量的存储和释放。
钠离子电池的工作原理主要包括充电和放电两个过程。
在充电过程中,钠离子从正极(如钠离子化合物)脱嵌,经由电解液传输至负极(如碳材料),同时伴随着电子的流动,形成钠金属和碳材料之间的锂离子化合物。
而在放电过程中,则是反过来的过程,钠离子从负极脱嵌,通过电解液传输至正极,同时释放出电子,完成能量的释放。
3. 钠离子电池的正负极材料正负极材料是钠离子电池中的关键组成部分,直接影响着电池的能量密度和循环寿命。
常用的正极材料包括氧化物、磷酸盐和硫化物等,而负极材料主要有碳材料和金属钠。
选择适合的正负极材料可以提高钠离子电池的能量密度和循环寿命。
4. 电解液的优化电解液是钠离子电池中的重要组成部分,主要起着传导离子和电子的作用。
优化电解液的组成可以提高钠离子电池的离子传输速率和电池性能。
目前,常用的电解液包括有机电解液和固态电解液两种类型。
有机电解液具有传导性好、电池成本低等优势,而固态电解液具有安全性好、循环寿命长等优势。
5. 钠离子电池性能的提升钠离子电池的性能主要包括能量密度、功率密度、循环寿命和安全性等方面。
为了提高钠离子电池的性能,研究人员采取了多种策略,包括优化正负极材料、改变电解液组成、改善电池结构等。
钠电池 电解液 半电池
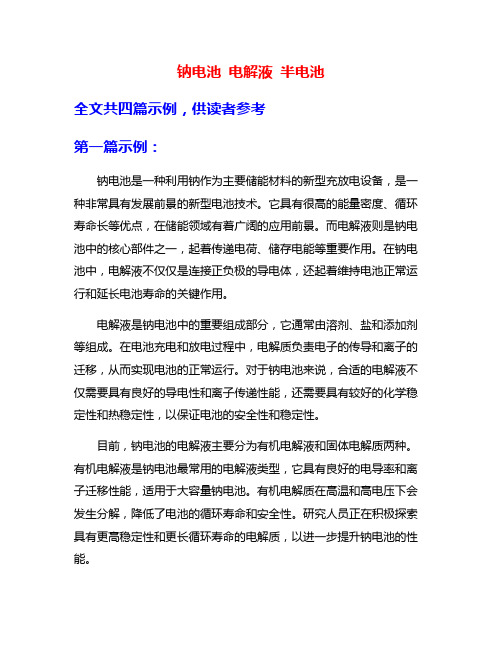
钠电池电解液半电池全文共四篇示例,供读者参考第一篇示例:钠电池是一种利用钠作为主要储能材料的新型充放电设备,是一种非常具有发展前景的新型电池技术。
它具有很高的能量密度、循环寿命长等优点,在储能领域有着广阔的应用前景。
而电解液则是钠电池中的核心部件之一,起着传递电荷、储存电能等重要作用。
在钠电池中,电解液不仅仅是连接正负极的导电体,还起着维持电池正常运行和延长电池寿命的关键作用。
电解液是钠电池中的重要组成部分,它通常由溶剂、盐和添加剂等组成。
在电池充电和放电过程中,电解质负责电子的传导和离子的迁移,从而实现电池的正常运行。
对于钠电池来说,合适的电解液不仅需要具有良好的导电性和离子传递性能,还需要具有较好的化学稳定性和热稳定性,以保证电池的安全性和稳定性。
目前,钠电池的电解液主要分为有机电解液和固体电解质两种。
有机电解液是钠电池最常用的电解液类型,它具有良好的电导率和离子迁移性能,适用于大容量钠电池。
有机电解质在高温和高电压下会发生分解,降低了电池的循环寿命和安全性。
研究人员正在积极探索具有更高稳定性和更长循环寿命的电解质,以进一步提升钠电池的性能。
除了电解液外,半电池也是钠电池中的重要组成部分。
半电池是一种带有正极和负极的独立结构,由正极材料、负极材料和电解质等组成。
正极材料通常采用氧化物、硫化物等物质,而负极材料则一般选择金属钠或碳材料。
在充放电过程中,半电池中的正极和负极相互转化,实现电子和离子的传递,从而完成能量储存和释放。
钠电池的发展受到了诸多因素的制约,如电解液的稳定性和半电池中的材料选择等。
未来,随着钠电池技术的不断进步和完善,相信钠电池将在储能领域展现出更广阔的应用前景。
我们也期待在电解液和半电池等关键技术领域取得新的突破,为钠电池的商业化应用提供更多可能性。
钠电池、电解液和半电池是构成钠电池系统的重要组成部分。
钠电池具有高能量密度、长循环寿命等优势,是一种具有巨大发展潜力的新型储能技术。
钠离子电池正极材料P2型NaxMnO2的制备和电化学性能研究

太原理工大学硕士研究生学位论文钠离子电池正极材料P2型Na x MnO2的制备和电化学性能研究摘要锂离子电池在便携电子设备、电动汽车等领域已经得到了广泛的应用,并且取得了巨大的成功且增长势头十分迅猛。
然而,锂资源储量较低导致锂离子电池成本不断升高,钠离子电池有望在大规模储能设备方面取代锂离子电池。
在众多钠离子电池正极材料中,层状过渡金属氧化物特别是钠锰氧化物具有高比容量和工作电压、易于制备、环境友好无毒性、成本低等优点得到了研究者广泛的关注,此外,与O3相结构相比,P2相结构具有较高的离子导电性和较低的扩散势垒。
因此,P2型锰基层状氧化物是一种极具潜力的钠离子电池正极材料。
本文探究了不同高温反应温度和不同高温反应时间及Cu2+掺杂对P2-Na0.67MnO2材料的影响;制备具有双极性特性的P2-Na0.5Ni0.25Mn0.75O2材料,对其进行Ti4+掺杂改性,并进一步研究Ti4+掺杂对Na0.5Ni0.25Mn0.75O2结构、形貌、电化学性能以及离子扩散速率的影响。
本论文主要研究结果如下:(1) 在采用高温固相法制备P2-Na0.67MnO2的过程中,不同的高温反应温度制备的材料晶格结构没有明显改变,其中温度在900℃时合成材料的结晶度最好,不同温度下合成材料的形貌均为微米级块状并存在一定程度的团聚现象。
900℃和1000℃合成的材料具有相似的高于150 mAh g-1的可逆容量,但是900℃制备的材料循环性能更好,100周容量保持率为78.1%,并且倍率性能明显优于1000℃合成的材料。
(2) 在900℃不同高温反应时间内,随着反应时间的延长,材料放电容量逐渐增大,当高温反应时间为15h时电化学性能最佳,首周放电容量可达164.8 mAh g-1,循环100周容量保持率为84.7%。
当反应时间过长达到I太原理工大学硕士研究生学位论文20h时,材料中会产生MnO2杂质并且放电容量会降低。
(3) Cu2+掺杂明显改变了材料的晶格结构,材料由正交晶系转变为六方晶系,掺杂量过高时会产生CuO杂质,但是掺杂之后材料形貌并没有明显的改变,仍然是微米级块状形貌。
钠电池 电解液 半电池

钠电池电解液半电池全文共四篇示例,供读者参考第一篇示例:钠电池是一种新型的电池技术,采用钠金属作为正极材料,相较于传统的锂电池具有更高的能量密度和循环寿命。
而电解液是钠电池中至关重要的一部分,它负责将正极和负极之间的电荷传递,从而实现电池的充放电过程。
半电池则是指仅包含正极或负极的电池,用以研究单个极性的电极材料的性能。
电解液在钠电池中起着至关重要的作用,它不仅负责导电和传递电荷,还影响电池的性能和循环寿命。
目前,研究者们探索了多种类型的电解液,包括无机电解液、聚合物电解质和固态电解质等。
固态电解质由于其高离子传导性和化学稳定性备受关注,有望解决传统液态电解液的安全性和稳定性问题。
固态电解质仍面临着制备工艺复杂和成本较高的挑战,限制了其在钠电池中的商业化应用。
在钠电池的发展过程中,半电池的研究扮演着重要的角色。
通过制备单个极性的半电池,研究者可以深入探究正负极材料的性能及其影响因素,为设计高性能的全电池提供依据。
半电池的研究也为解决电池中的材料失效问题提供了新的思路,可实现对正负极材料的独立优化,从而提高电池的循环寿命和能量密度。
钠电池、电解液和半电池是钠储能技术研究中的重要组成部分,它们共同推动着钠电池技术的不断进步。
随着材料科学和能源储存技术的发展,钠电池有望成为未来能源系统中的重要组成部分,为实现清洁、可持续的能源供应做出贡献。
希望未来能够有更多的研究和创新,推动钠电池技术的商业化应用,为构建绿色能源体系做出贡献。
第二篇示例:钠电池是一种使用钠作为主要正极材料的电池。
在钠电池中,电解液扮演着重要的角色,它不仅负责媒介正负极之间的离子传输,还对电池的性能和寿命起着关键作用。
本文将重点讨论钠电池中的电解液及其对半电池的影响。
一、钠电池的基本原理钠电池是一种二次电池,其工作原理与锂电池类似,都是通过正负极材料的电化学反应来完成电荷与放电过程。
在充电过程中,正极材料(如氧化钠)释放出氧离子,负极材料(如钠)吸收氧离子,同时放出电子,将电子通过外部电路传递到正极,使氧离子还原成氧气,完成电荷过程。
钠电池电解液
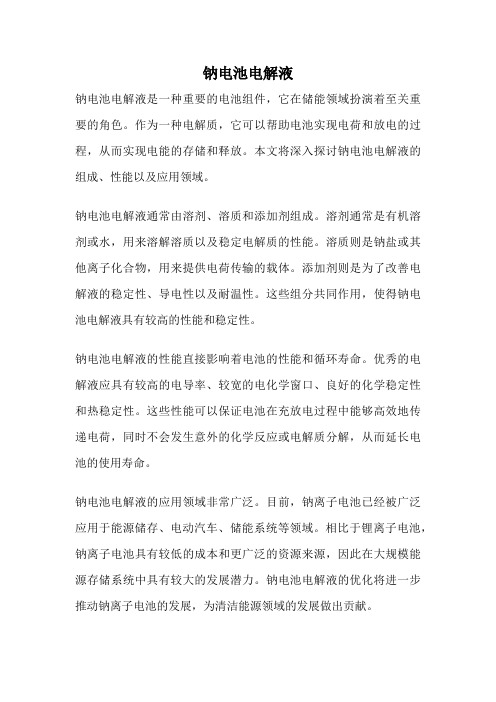
钠电池电解液
钠电池电解液是一种重要的电池组件,它在储能领域扮演着至关重要的角色。
作为一种电解质,它可以帮助电池实现电荷和放电的过程,从而实现电能的存储和释放。
本文将深入探讨钠电池电解液的组成、性能以及应用领域。
钠电池电解液通常由溶剂、溶质和添加剂组成。
溶剂通常是有机溶剂或水,用来溶解溶质以及稳定电解质的性能。
溶质则是钠盐或其他离子化合物,用来提供电荷传输的载体。
添加剂则是为了改善电解液的稳定性、导电性以及耐温性。
这些组分共同作用,使得钠电池电解液具有较高的性能和稳定性。
钠电池电解液的性能直接影响着电池的性能和循环寿命。
优秀的电解液应具有较高的电导率、较宽的电化学窗口、良好的化学稳定性和热稳定性。
这些性能可以保证电池在充放电过程中能够高效地传递电荷,同时不会发生意外的化学反应或电解质分解,从而延长电池的使用寿命。
钠电池电解液的应用领域非常广泛。
目前,钠离子电池已经被广泛应用于能源储存、电动汽车、储能系统等领域。
相比于锂离子电池,钠离子电池具有较低的成本和更广泛的资源来源,因此在大规模能源存储系统中具有较大的发展潜力。
钠电池电解液的优化将进一步推动钠离子电池的发展,为清洁能源领域的发展做出贡献。
总的来说,钠电池电解液作为钠离子电池的重要组成部分,具有重要的意义和应用前景。
通过不断优化电解液的配方和性能,将有助于提高钠离子电池的能量密度、循环寿命和安全性,推动清洁能源技术的发展。
希望未来钠电池电解液能够得到更多的研究和应用,为人类社会的可持续发展做出更大的贡献。
- 1、下载文档前请自行甄别文档内容的完整性,平台不提供额外的编辑、内容补充、找答案等附加服务。
- 2、"仅部分预览"的文档,不可在线预览部分如存在完整性等问题,可反馈申请退款(可完整预览的文档不适用该条件!)。
- 3、如文档侵犯您的权益,请联系客服反馈,我们会尽快为您处理(人工客服工作时间:9:00-18:30)。
In search of an optimized electrolyte for Na-ion batteriesAlexandre Ponrouch,*ac Elena Marchante,ac Matthieu Courty,bc Jean-Marie Tarascon bc and M.Rosa Palac ın *acReceived 17th May 2012,Accepted 12th June 2012DOI:10.1039/c2ee22258bElectrolytes are essential for the proper functioning of any battery technology and the emerging Na-ion technology is no exception.Hence,a major focus on battery research is to identify the most appropriate formulation so as to minimize interface reactions and enhance both cell performances and safety aspects.In order to identify suitable electrolyte formulations for Na-ion chemistry we benchmarked various electrolytes containing diverse solvent mixtures (cyclic,acyclic carbonates,glymes)and Na-based salts having either F-based or perchlorate anions and measured viscosity,ionic conductivity,and thermal and electrochemical stability.The binary EC:PC solventmixture has emerged as the best solvent formulation and has been used to test the performance of Na/hard carbon cells with both NaClO 4and NaPF 6as dissolved salts.Hard carbon electrodes having reversible capacities of 200mA h g À1with decent rate capability and excellent capacity retention (>180cycles)were demonstrated.Moreover,DSC heating curves demonstrated that fully sodiated hard carbon cycled in NaPF 6–EC:PC exhibits the highest exothermic onset temperature and nearly the lowest enthalpy of reaction,thus making this electrolyte most attractive for the development of Na-ion batteries.1.IntroductionOwing to their success in the domain of portable electronics,lithium batteries are currently considered as the key technology for electric vehicle propulsion and in parallel are starting to be evaluated for stationary applications.However,the imple-mentation of a lithium-based technology for mass storage faces an important challenge linked to both lithium availability and cost.We face the risk that lithium resources cannot cope over thenext decades with the foreseen staggering energy storage demands dictated by both automotive transportation and grid-related applications.In anticipation of such a scenario,besides promoting recycling,new sustainable chemistries must be developed,and the most appealing alternative is to use sodium instead of lithium.There are several reasons:the intercalation chemistry is similar to that of lithium,sodium resources are in principle unlimited and it is an element easy to recycle.Sodium technology has already been successfully implemented in high temperature Na/S cells for MW storage and Na/NiCl 2ZEBRA-type systems for electric vehicles,both of which take advantage of the highly conducting solid beta-alumina ceramics at temperatures of ca.300 C.Mindful of these considerations,within the current knowledge gained in Li-ion technology,a room temperature Na-ion cell should be feasible and is a realisticaInstitut de Ci encia de Materials de Barcelona,ICMAB-CSIC,Campus de la UAB,08193Bellaterra,Spain.E-mail:rosa.palacin@icmab.es;aponrouch@icmab.es;Fax:+34935805729;Tel:+34935801853#279bLaboratoire de R eactivit e et Chimie des Solides,Universit e de Picardie Jules Verne,CNRS UMR7314,33rue Saint Leu,80039Amiens,France cALISTORE-ERI European Research Institute,Dynamic Article Links CEnergy &Environmental ScienceCite this:Energy Environ.Sci.,2012,5,/eesPAPERD o w n l o a d e d b y S h a n g h a i I n s t i t u t e o f C e r a m i c s , C A S o n 07 S e p t e m b e r 2012P u b l i s h e d o n 13 J u n e 2012 o n h t t p ://p u b s .r s c .o r g | d o i :10.1039/C 2E E 22258BView Online / Journal Homepage / Table of Contents for this issuetarget.This technology would be inherently safer than the high temperature cells that contain molten sodium and corrosive liquids and would not suffer from long start up times (issued from the need to warm these cells slowly to their operation temperatures).While sodium-based cells will always fall short of matching the specific energy of the corresponding lithium tech-nology at the cell level,they are certainly an option for large scale applications in which cost instead of energy density is the over-riding factor.1,2Historically,progress in sodium intercalation chemistry is parallel to that of lithium.3,4Therefore,Na-based systems rapidly fall into oblivion due to the rapid advent and success of the lithium ion technology for portable electronics and of its positive attributes for a wide range of applications requiring energy densities from m W h to MW h.However,for sustainability reasons the Na-ion concept,which requires both positive and negative Na-based insertion compounds,has recently re-captured the scientific community’s attention.5–10While a wide variety of Na-based positive electrodes have been reported,11–21hard carbon constitutes presently the most viable option for the negative electrode.When it comes to transition metal oxides,the choice is rather limited owing to the competi-tion between insertion vs.conversion 22reactions at low poten-tials,the former being only favoured for early 3d metal oxides.While Na x VO 2has been proved to reversibly react with sodium at moderate potentials,23,24we recently identified Na 2Ti 3O 7as an effective low potential insertion sodium compound.Indeed,it exhibits the reversible uptake of 2Na ions per formula unit (200mA h g À1)at an average potential of 0.3V vs.Na +/Na 0through a two-phase redox mechanism.25,26The cycling of this electrode vs.Na +/Na 0is still problematic due to a poorly stable electrode/electrolyte interphase (SEI)and calls for further studies to elucidate both the structural changes concomitant to the redox processes and the complex growth mechanism of the SEI together with its composition and nature.While a number of efforts are being directed to the search for new electrode materials for sodium ion batteries,studies dealing with the electrolyte are much scarcer.Still,some available reports demonstrate that the SEI formed on carbonaceous electrodes is markedly different for sodium-and lithium-based electrolytes even on using the same solvent.5,27Moreover,vinylene carbonate (VC),a common additive in Li-based electrolytes,appears to be detrimental to the performance of Na cells.28Presently,most studies are being performed using NaClO 4in PC as the electro-lyte with only a few reports on the use of NaPF 6and other solvents,5,27,29but no systematic studies have been reported on their physico-chemical properties.Yet,this is a must if we ever want the Na-ion technology to become a commercial reality and a serious contender for electrochemical storage.As in any other battery system,a good electrolyte should exhibit:(i)good ionic conductivity,(ii)a large electrochemical window (i.e.,high and low onset potential for electrolyte decomposition through oxidation and reduction at high and low voltages,respectively),(iii)no reactivity towards the cell components,and (iv)a large thermal stability window (i.e.melting point and boiling point lower and higher than the stan-dard temperatures for the cell utilization,respectively).Finally,it should be intrinsically safe,have as low toxicity as possible and meet cost requirements for the targeted applications.All thesefeatures are intrinsically dependent on the nature of the salt and the solvent(s)and the possible use of additives.To address the aforementioned issues we embarked on a comparative survey of electrolytes prepared using several solvents (PC,EC,DMC,DME,DEC,THF and Triglyme)and solvent mixtures (EC:DMC,EC:DME,EC:PC and EC:Tri-glyme)in combination with different Na salts,namely NaClO 4,NaPF 6and NaTFSI.This paper is mainly divided into two parts.The first part deals with the intrinsic physico-chemical properties of electrolytes:viscosity,ionic conductivity and electrochemical and thermal stability,while the second part addresses their low voltage electrochemical performance using hard carbon (HC)as the electrode plementary differential scanning calorimetry (DSC)and electrochemical impedance spectroscopy (EIS)analysis were performed in the hope of grasping some information about the SEI growth and thermal stability.2.Experimental section2.1.Electrolyte preparationAll tested electrolytes consist of a 1M solution of the salt (NaClO 4,NaPF 6or NaTFSI)in propylene carbonate (PC hereafter,Aldrich,anhydrous,99.7%),ethylene carbonate (EC,Aldrich,anhydrous,99.0%),dimethyl carbonate (DMC,Aldrich,anhydrous,99.0%),diethyl carbonate (DEC,Aldrich,anhydrous,99.0%),dime-thoxyethane (DME,Aldrich,anhydrous,99.5%),tetrahydrofuran (THF,Aldrich,anhydrous,99.9%)and triethylene glycol dimethyl ether (Triglyme,Aldrich,99.0%)or binary solvent mixtures (50/50wt%).Solvents were used as received.Salts were vacuum dried at moderate temperatures prior to being used.The water content in all as-prepared electrolytes was measured by Karl-Fisher titration and found to be lower than 50ppm in all cases.Thus,they were used without any additional drying step.2.2.Conductivity and viscosity measurementsThe ionic conductivity of the electrolytes was measured with an Oakton CON 11standard conductivity meter.Their viscosities were measured with a RheoStress RS600Rheometer (HAAKE)at 20 C.All measurements were done at room temperature and were performed simultaneously in order to avoid any bias due to changes in ambient conditions.2.3.Differential scanning calorimetryDSC measurements were performed using a DSC 204F1Netzsch calorimeter,with a heating rate of 10 C min À1.The sample,either solid or liquid,was placed in an aluminum capsule which was sealed in a dry glove box filled with argon and pierced once inside the DSC apparatus.Melting points of the solvents and the electrolytes were measured from the DSC heating curves after cooling the sample down to À120 C,using liquid nitrogen.Boiling points and phase transition temperatures were assessed by heating the sample up to 350 C.Thermal stability and heat generated from fully sodiated HC were measured from electrodes reduced to 3mV vs.Na +/Na 0at C /40,and recovered inside the argon filled dry box without any washing or drying.Afterwards,the samples were placed in stainless steel capsules which were not pierced.D o w n l o a d e d b y S h a n g h a i I n s t i t u t e o f C e r a m i c s , C A S o n 07 S e p t e m b e r 2012P u b l i s h e d o n 13 J u n e 2012 o n h t t p ://p u b s .r s c .o r g | d o i :10.1039/C 2E E 22258B2.4.ElectrochemistryThe electrochemical stability of the electrolytes was evaluated inthree-electrode Swagelok cells 30using a sodium metal cube slice (Aldrich,99.95%)as counter and reference electrodes,and an aluminum plunger as the working electrode (WE).The standard cycling protocol consists of three subsequent steps hereafter called Protocol A,B and C (cf.Fig.1).Protocol A is mainly aimed at determining the electrolyte electrochemical stability window,via the collection of CV curves at a sweep rate of 10mV s À1and of EIS spectra prior and after each CV (denoted EIS-A1,EIS-A2and EIS-A3).The first CV is carried out between 0.01and 3V vs.Na +/Na 0in order to evaluate the electrochemical stability of the electrolyte at low potentials.In subsequent CVs,the potential window is being enlarged every 3cycles to gradually attain 5.3V vs.Na +/Na 0with 400mV steps.Protocol B is mainly geared towards the determination of possible electrolyte decomposition upon various cycling conditions via EIS measurements which were performed between several linear polarization (LP)sequences (at a 10mV s À1sweep rate).Typically,the WE potential was left at the open circuit potential (OCP)for at least 2h after protocol A,and EIS-B1was recorded.Then,several LP steps were applied to the WE with EIS measurements between them (denoted as EIS-B2up to EIS-B7).These were made at three different potential values,namely 0.01,3and 5V vs.Na +/Na 0.Protocol C is devoted to WE performance when the potential is being held either at high (5V vs.Na +/Na 0)or low (0.01V vs.Na +/Na 0)values for various periods of time.More specifically,the WE was left at OCP for at least 2h after protocol B,and EIS-C1was measured as to establish an initial state of the electrode.Then a LP (at a 10mV s À1sweep rate)up to 5V vs.Na +/Na 0was applied,the potential being held at 5V vs.Na +/Na 0for various periods of time (ca.30min,1h 30min,3h and 5h)between which EIS measurements were performed (ca.EIS-C2up to EIS-C6).Finally,LP (at a 10mV s À1sweep rate)down to 0.01V vs.Na +/Na 0was applied and the potential was held at 0.01V vs.Na +/Na 0for ca.30min,1h 30min,3h and 5h,between which EIS measurements were also performed (EIS-C7up to EIS-C11).EIS measurements (10mV perturbation amplitude,with frequencies ranging from 1000kHz to 50mHz)were also per-formed upon cycling of HC tape-cast electrodes using three-electrode Swagelok cells (after a 2h OCP period).Cycling experiments were also performed in two-electrode Swagelok cells in galvanostatic mode with potential limitation (GCPL)at different rates ranging from C /20to C (1C being one Li +inserted in one hour)to monitor capacity evolution upon cycling.All electrochemical tests were performed using a Bio-Logic VMP3potentiostat and twin cells were assembled in order to ensure reproducibility of results.Hard carbon (HC)was prepared following the procedure described in ref.31by pyrolysis of sugar at 1100 C for 6h under argon flposite electrodes mimicking industrial technol-ogies were prepared from slurries (90wt%HC,5wt%poly-vinylidene fluoride binder (PVDF,Arkema)and 5wt%Super P carbon (Csp hereafter from Timcal)in N -methylpyrrolidoneFig.1Schematic representation of the standard electrochemical protocols applied for electrolyte electrochemical characterization in three-electrode Swagelok cells.D o w n l o a d e d b y S h a n g h a i I n s t i t u t e o f C e r a m i c s , C A S o n 07 S e p t e m b e r 2012P u b l i s h e d o n 13 J u n e 2012 o n h t t p ://p u b s .r s c .o r g | d o i :10.1039/C 2E E 22258B(NMP,Aldrich)).Mixing was done using either a PM100Retsch Planetary Ball Miller (stainless steel container with 3stainless steel balls of 1cm diameter at 500rpm for 2h with change of the rotating way every 30min)or a high energy SPEX 800ball miller for 30min,the latter resulting in higher capacities.These slurries were tape-cast on a 20m m thick copper foil (Goodfellow)with a 250m m Doctor-Blade and further dried at 120 C under vacuum.Once dried,0.8cm 2disk electrodes were cut and pressed at 8t prior to testing.Typically,electrode loading is 2mg corre-sponding to about ca.15m m thick deposits.3.Results and discussion3.1.Conductivity and viscosity measurementsThe electrolyte ionic conductivity is one of the most crucial factors for cell performance as it ‘‘defines how fast the energy can be released by controlling the rate of mass flow within the battery’’.32It depends on several factors,such as (i)the degree of dissociation of the salt (which is dictated by the dielectric constant of the solvent),(ii)the viscosity of the electrolyte (which influences the ion mobility),and (iii)the transport number of the Na +cation and its counter anion.The ionic conductivity values for the investigated electrolytes are reported in Fig.2and benchmarked against LP30(1M LiPF 6in EC:DMC),which is presently the most widely used electrolyte for lithium ion cells.To separate the relative influences of the solvent and the salt,PC was chosen as the solvent and the conductivities of 1M solutions of different salts were evaluated,and afterwards the conductiv-ities of 1M NaClO 4in different solvent mixtures were measured.Each reported value here is an average of three measurements,the deviation between them being less than 5%,which gives us confidence that our trends are robust.The conductivity values for PC-based electrolytes (cf.Fig.2a)are relatively similar,ranging from 7.98for NaPF 6to 6.2mS cm À1for NaTFSI and being intermediate for NaClO 4.This dependence upon the nature of the anion is consistent with what was previously reported for Li-based electrolytes 33which was ascribed to the lower polarizing character of the PF 6Àanions,which would improve salt dissociation and enhance ionic mobility (see ref.32,p.4315).In contrast,larger ionic conduc-tivity variations were found by fixing the salt (NaClO 4)and changing the nature of the solvents with s values following the trend EC:DME >EC:DMC >EC:PC >EC:Triglyme >EC:DEC >PC >Triglyme \DME,DMC,DEC (Fig.2b).These results confirm that single solvent-based electrolytes show much lower conductivity than binary solvent-based elec-trolytes,this being true even for PC,which presents a very high dielectric constant value of 64.92.The addition of EC as a co-solvent strongly improved the ionic conductivity in all cases.The lowest ionic conductivity for binary mixtures is measured for EC:DEC (ca. 6.35mS cm À1)and the highest for EC:DMEFig.2Conductivity (black bars and left hand side y axis)and viscosity (green bars and right hand side y axis)values of (a)PC based electrolytes with 1M of various Na salts and (b)electrolytes based on 1M NaClO 4dissolved in various solvents and solvent mixtures.D o w n l o a d e d b y S h a n g h a i I n s t i t u t e o f C e r a m i c s , C A S o n 07 S e p t e m b e r 2012P u b l i s h e d o n 13 J u n e 2012 o n h t t p ://p u b s .r s c .o r g | d o i :10.1039/C 2E E 22258B(ca.12.55mS cm À1),which is fully comparable to LP30(dashed line in Fig.2b).Such differences in the values of ionic conduc-tivity were found to be proportional to the dielectric constant of the EC co-solvent (PC >Triglyme >DME >DMC >DEC,see Table 1).The only exceptions to this trend are PC and Triglyme-based electrolytes which present lower ionic conductivity,most certainly due to their higher viscosity values as compared to other co-solvents (PC >Triglyme >DEC >DMC >DME,seeTable 1).As a conclusion,the nature of the co-solvent can enhance ionic conductivity through improving the dissociation of the salt (if the dielectric constant is high)and/or by lowering the viscosity of the resulting electrolyte and thus improving the ionic mobility.Viscosity measurements were performed in order to better understand this issue and results are displayed in Fig.2.Similar values of about 6.8cP are observed for PC-based electrolytes with 1M NaTFSI,NaClO 4or NaPF 6(cf.Fig.2a),in agreement with the nature of the counter anions not having a significant effect on the viscosity.On comparing the values measured for various solvents and solvent mixtures with 1M NaClO 4(cf.Fig.2b)we can see that the viscosity of single solvent-based electrolytes (i.e.1M NaClO 4in DMC,Triglyme or PC)follows the same trend as for the solvent alone (i.e.PC >Triglyme >DMC,see Table 1and Fig.2b).Viscosities for pure DMC,Triglyme and PC are 0.5,1.6and 2.2cP,respectively;while higher values are recorded for 1M NaClO 4solutions (ca.0.65,5.8and 6.8cP,respectively).The same observation is made for binary solvent EC-based electrolytes (with 1M NaClO 4),their viscosities being proportional to that of the co-solvent,thus following the trend:EC:PC >EC:Triglyme >EC:DEC >EC:DMC >EC:DME (cf.Fig.2b).Amongst single solvent-based electrolytes (i.e.1M NaClO 4in DMC,Triglyme or PC)the highest ionic conductivity and the highest viscosity are both observed for PC,which confirms that dissociation of the salt is the key parameter (i.e.high dielectric constant value).In contrast,for binary solvent-based electrolytes (i.e.1M NaClO 4in EC:PC,EC:Triglyme,EC:DEC,EC:DMC or EC:DME)the highest ionic conductivity is recorded for EC:DME even though DME does not exhibit the highest dielectric constant value (cf.Table 1),yet it presents the lowest viscosity among all binary solvent-based electrolytes (cf.Fig.2b).This confirms that ion mobility is the most important factor here (i.e.low viscosity value),the presence of EC already allowing good dissociation of the salt.3.2.Differential scanning calorimetry measurementsMelting point is a common issue for EC-based electrolytes in lithium ion batteries,since it determines the operatingtemperature range.Although EC presents a very high dielectric constant (ca.89.78),which enables a high dissociation of the salt and is recognized to be key in the formation of a stable SEI on the most common anode in lithium ion cells (graphite),its main drawback is nested in its relatively high melting point (ca.36.4 C).Such temperature can of course be lowered by the addition of the salt,but the changes are not sufficient for battery applications.This is the reason why over the years,numerous co-solvents were added to EC in the quest to lower the melting point of electrolytes.34,35Within this context,we performed DSC measurements on the various Na-based electrolytes studied herein in order to determine their solidification temperature and hence their temperature utilization range.The DSC curves for electrolytes previously cooled down to À120 C and then heated up to room temperature at a scan-ning rate of 10 C min À1are shown in Fig.3.They significantly differ from one binary EC-based solvent to another as witnessed by the temperature of the first endothermic peak,related to the first crystallization,which shifts from À25,À50to À75 C for DMC,DEC and DME co-solvents,respectively.Interesting and somewhat puzzling is the absence of an endothermic peak in the DSC heating curve of the PC-based electrolyte.We could solely spot a vitreous transition for temperatures near À95 C.In short,no electrolyte solidification is observed when PC is added as the co-solvent,which is ofTable 1Dielectric constants and measured viscosities of the solvents Solvents Dielectric constant Viscosity (cP)EC 89.78##PC64.92 2.2Triglyme 7.5 1.6DME 7.20.7DMC 3.1070.5DEC2.8050.3Fig.3DSC heating curves of electrolytes after cooling the sample down to À120 C.(a)Electrolytes based on 1M NaClO 4dissolved in various solvent mixtures and (b)PC based electrolytes with 1M of various Na salts.D o w n l o a d e d b y S h a n g h a i I n s t i t u t e o f C e r a m i c s , C A S o n 07 S e p t e m b e r 2012P u b l i s h e d o n 13 J u n e 2012 o n h t t p ://p u b s .r s c .o r g | d o i :10.1039/C 2E E 22258Bprimary importance for low temperature application since the electrolyte remains in liquid form at very low temperatures behaving seemingly like ionic liquid-based electrolytes.36This does not come as a total surprise as PC is frequently used as a co-solvent in Li-ion battery electrolytes to lower their operating temperatures.The vitreous transition is observed for all PC-based electrolytes studied in this work (cf.Fig.3b),whatever the nature of the salts,and was never reported to our knowledge for Li-based electrolytes.This finding is somewhat intriguing and efforts are currently underway to understand the origin of this phenomenon.The thermal stability of the solvents and electrolytes at high temperature was also evaluated by heating from room temper-ature to 350 C (Fig.4).Two different endothermic peaks are observed for EC:DME;EC:DMC and EC:DEC mixtures without any sodium salt (cf.Fig.4b)with onset temperatures equal to that of each solvent heated separately (cf.Fig.4a).This indicates that no eutectics are formed within the composition ranges studied.Yet,the EC:PC mixture shows a single exothermic peak around 250 C with the highest thermal stability amongst all the solvent combinations that we have tested.Similar DSC heating curves were recorded for EC:DEC alone and 1M NaClO 4in an EC:DEC electrolyte,the onset temper-ature for the endothermic peak (Fig.4d)being slightly shifted to lower temperatures as expected.The additional tiny endothermic peak appearing at ca.310 C corresponds to the melting point of the salt after evaporation of the solvents as confirmed by DSC experiments performed on salts alone (Fig.4c),which addition-ally indicate that amongst the various Na _based salts NaClO 4is the most thermally stable:NaClO 4(310 C)>NaPF 6(280 C)>NaTFSI (250 C).A similar thermal stability trend was reported for the homologous Li-based salts as a function of the anion withLiClO 4(236 C)being more stable than LiPF 6(200 C)and LiTFSI (234 C),and therefore a lower overall thermal stability owing to their lower Magdelung energy as compared to that of the Na-based salts.3.3.Electrochemical potential windowElectrochemical tests were performed using three-electrode Swagelok cells with Na as counter and reference electrodes.Only high purity aluminum plungers,free of lead,could be used as the working electrode to probe a wide potential window (0to 5.3V),otherwise the formation of Na 15Pb 4(cf.Fig.5)is observed.37The CVs collected for (i)single solvent based electrolytes with 1M NaClO 4,(ii)binary solvent based electrolytes with 1M NaClO 4,and (iii)PC with different salts are reported in Fig.6a–f,respectively.Out of them,three electrolyte combinations stand out as exhibiting poorer electrochemical stability than the others either upon oxidation or reduction.Indeed,a high reduction current is measured for 1M NaClO 4in EC:Triglyme with an onset potential of ca.1.1V vs.Na +/Na 0(cf.Fig.6b)while important oxidation currents are recorded for 1M NaClO 4in THF and 1M NaTFSI in PC with onset potentials of ca.4.26V and 3.3V vs.Na +/Na 0,respectively (cf.Fig.6a and c).In the latter case the observed oxidation current most likely corre-sponds to the well-known corrosion of the Al plunger in contact with TFSI based electrolytes.38This issue can be alleviated by adding a small quantity of NaPF 6(5wt%)to the electrolyte as previously reported in the case of Li-based electrolytes 39(see Fig.6f).When dealing with the remaining single solvent based elec-trolytes with 1M NaClO 4,the electrochemical stability (Fig.6d)decreases following the trend DEC >PC >Triglyme >DME >Fig.4DSC heating curves up to 350 C of (a)solvents alone,(b)solvent mixtures,(c)PC based electrolytes with 1M of various Na salts and (d)EC:DEC solvent mixture with or without a sodium salt (1M NaClO 4).D o w n l o a d e d b y S h a n g h a i I n s t i t u t e o f C e r a m i c s , C A S o n 07 S e p t e m b e r 2012P u b l i s h e d o n 13 J u n e 2012 o n h t t p ://p u b s .r s c .o r g | d o i :10.1039/C 2E E 22258BDMC >THF.Under similar experimental conditions,the elec-trochemical stability of binary solvent-based electrolytes (cf.Fig.6e)decreases following the trend EC:PC >EC:DMC >EC:DME >EC:DEC >EC:Triglyme (Fig.6e).The electro-chemical stability trend of EC-based binary solvents thus differs from the one observed for the co-solvent alone.This indicates,as already pointed out by Tarascon and Guyomard,33that the electrochemical stability of a solvent mixture does not strictly depend on that of each co-solvent since synergetic effects do occur when solvents are mixed.Finally,for a defined solvent mixture,similar results were observed independent of the nature of the salt (Fig.6f).The electrochemical stability window was further studied by performing EIS measurements according to Protocols B and C defined in our standard characterization procedure (cf.Fig.1).Similar trends are observed for all electrolytes tested,and the results obtained for 1M NaClO 4–EC:DMC are shown in Fig.7.The Nyquist plot obtained for Protocol B with EIS measure-ments at 5V vs.Na +/Na 0is similar to the one obtained before any polarization at the OCP (cf.Fig.7a).In contrast,the Nyquist plots for EIS measurements at 0.01V vs.Na +/Na 0present a growing charge transfer resistance contribution upon cycling (semi-circular feature at high frequencies).This appears together with a smaller Warburg diffusion tail.These features are char-acteristic of the growth of a passivation layer (SEI)at the surface of the working electrode.40The fact that these features dis-appeared at each potential sweep of up to 5V vs.Na +/Na 0is consistent with the concomitant disappearance of this SEI layer at this potential.These observations are further confirmed by the EIS measurements within Protocol C,where the potential was held at either 5V or 0.01V vs.Na +/Na 0(cf.Fig.7b and c,respectively).No change was observed when the potential was held at 5V vs.Na +/Na 0(cf.Fig.7b)whereas the growth of the SEI layer at 0.01V vs.Na +/Na 0is clearly observable from the appearance of the above-mentioned characteristic features on the Nyquist plots (cf.Fig.7c).It is also worth mentioning that a fast decrease in the reduction current intensity is observed upon cycling at low potential values (cf.Fig.5b),confirming the formation of a passivation layer which limits the electrolyte reactivity at the WE interface.3.4.Electrolyte selectionFig.8depicts the electrochemical stability window for all elec-trolyte formulations studied,which,as mentioned above,isFig.5CVs (10mV s À1,1M NaClO 4in PC)obtained in three-electrode Swagelok cells of a high purity Al plunger.Inset displays CVs obtained in similar conditions using low purity Al plunger containing lead impurities resulting in Na–Pb alloyformation.Fig.6CVs (10mV s À1,high purity Al plungers)obtained in three-electrode Swagelok cells with (a)1M NaClO 4dissolved in single solvents,(b)1M NaClO 4dissolved in solvent mixtures and (c)various salts (1M)in PC.(d),(e)and (f)are low current zoom plots of (a),(b)and (c),respectively.D o w n l o a d e d b y S h a n g h a i I n s t i t u t e o f C e r a m i c s , C A S o n 07 S e p t e m b e r 2012P u b l i s h e d o n 13 J u n e 2012 o n h t t p ://p u b s .r s c .o r g | d o i :10.1039/C 2E E 22258B。