Autothermal Reforming of Methane over Ni Catalysts Supported on CuO-ZrO2-CeO2-Al2O3
天然气蒸汽重整制氢技术研究现状
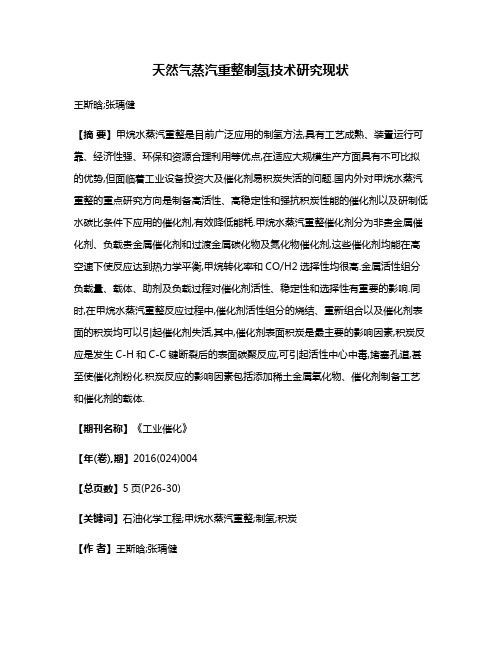
天然气蒸汽重整制氢技术研究现状王斯晗;张瑀健【摘要】甲烷水蒸汽重整是目前广泛应用的制氢方法,具有工艺成熟、装置运行可靠、经济性强、环保和资源合理利用等优点,在适应大规模生产方面具有不可比拟的优势,但面临着工业设备投资大及催化剂易积炭失活的问题.国内外对甲烷水蒸汽重整的重点研究方向是制备高活性、高稳定性和强抗积炭性能的催化剂以及研制低水碳比条件下应用的催化剂,有效降低能耗.甲烷水蒸汽重整催化剂分为非贵金属催化剂、负载贵金属催化剂和过渡金属碳化物及氮化物催化剂,这些催化剂均能在高空速下使反应达到热力学平衡,甲烷转化率和CO/H2选择性均很高.金属活性组分负载量、载体、助剂及负载过程对催化剂活性、稳定性和选择性有重要的影响.同时,在甲烷水蒸汽重整反应过程中,催化剂活性组分的烧结、重新组合以及催化剂表面的积炭均可以引起催化剂失活,其中,催化剂表面积炭是最主要的影响因素,积炭反应是发生C-H和C-C键断裂后的表面碳聚反应,可引起活性中心中毒,堵塞孔道,甚至使催化剂粉化.积炭反应的影响因素包括添加稀土金属氧化物、催化剂制备工艺和催化剂的载体.【期刊名称】《工业催化》【年(卷),期】2016(024)004【总页数】5页(P26-30)【关键词】石油化学工程;甲烷水蒸汽重整;制氢;积炭【作者】王斯晗;张瑀健【作者单位】东北石油大学,黑龙江大庆163714;中国石油石油化工研究院大庆化工研究中心,黑龙江大庆163714;中国石油石油化工研究院大庆化工研究中心,黑龙江大庆163714【正文语种】中文【中图分类】TE624.9+2;TQ426.95综述与展望CLC number:TE624.9+2;TQ426.95 Document code: A Article ID: 1008-1143(2016)04-0026-05H2具有热转化效率高、环境零污染、能量密度高和输送成本低等优点,是目前最具吸引力的清洁高效能源,在石油化工和动力燃料行业中均有广泛应用[1-2]。
甲烷自热重整反应器

甲烷自热重整反应器英文回答:Methane Autothermal Reforming Reactor.Methane autothermal reforming (ATR) is a process for converting methane into synthesis gas, a mixture of hydrogen and carbon monoxide. The process is typically carried out in a reactor that consists of a packed bed of catalyst particles. The catalyst is typically a nickel-based catalyst supported on a ceramic material. The methane feedstock is mixed with air or oxygen and preheated before entering the reactor. The reaction is exothermic, so the heat generated by the reaction is used to preheat the feedstock. The products of the reaction are synthesis gas, water, and carbon dioxide.The design of the ATR reactor is critical to the efficiency of the process. The reactor must be able to provide a uniform distribution of the reactants over thecatalyst surface, and it must be able to remove the heat generated by the reaction. The reactor must also be able to withstand the high temperatures and pressures that are required for the reaction to occur.There are a number of different reactor designs that can be used for ATR. The most common type of reactor is the shell-and-tube reactor. In this type of reactor, the catalyst particles are packed into a tube, and the reactants are passed through the tube. The heat generated by the reaction is removed by cooling water that flows through the shell of the reactor.Another type of reactor that is used for ATR is the fluidized bed reactor. In this type of reactor, thecatalyst particles are fluidized by a stream of gas. The reactants are passed through the fluidized bed of catalyst particles, and the heat generated by the reaction is removed by the gas stream.The design of the ATR reactor is a critical factor in the efficiency of the process. The reactor must be able toprovide a uniform distribution of the reactants over the catalyst surface, and it must be able to remove the heat generated by the reaction. The reactor must also be able to withstand the high temperatures and pressures that are required for the reaction to occur.中文回答:甲烷自热重整反应器。
Steam reforming of methanol over CuZnZrGa catalyst effect of the reduction conditions
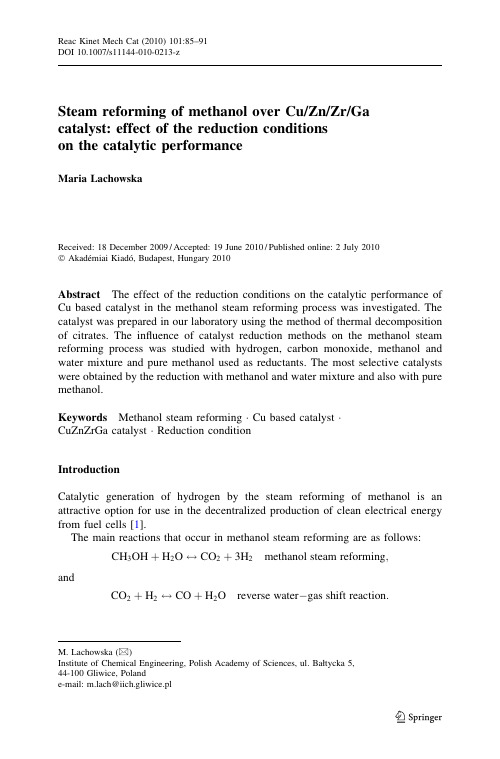
Reac Kinet Mech Cat(2010)101:85–91DOI10.1007/s11144-010-0213-zSteam reforming of methanol over Cu/Zn/Zr/Ga catalyst:effect of the reduction conditionson the catalytic performanceMaria LachowskaReceived:18December2009/Accepted:19June2010/Published online:2July2010ÓAkade´miai Kiado´,Budapest,Hungary2010Abstract The effect of the reduction conditions on the catalytic performance of Cu based catalyst in the methanol steam reforming process was investigated.The catalyst was prepared in our laboratory using the method of thermal decomposition of citrates.The influence of catalyst reduction methods on the methanol steam reforming process was studied with hydrogen,carbon monoxide,methanol and water mixture and pure methanol used as reductants.The most selective catalysts were obtained by the reduction with methanol and water mixture and also with pure methanol.Keywords Methanol steam reformingÁCu based catalystÁCuZnZrGa catalystÁReduction conditionIntroductionCatalytic generation of hydrogen by the steam reforming of methanol is an attractive option for use in the decentralized production of clean electrical energy from fuel cells[1].The main reactions that occur in methanol steam reforming are as follows: CH3OHþH2O$CO2þ3H2methanol steam reforming;andCO2þH2$COþH2O reverse waterÀgas shift reaction:chowska(&)Institute of Chemical Engineering,Polish Academy of Sciences,ul.Bałtycka5,44-100Gliwice,Polande-mail:ch@iich.gliwice.pl123chowska Many papers concerning the performance of Cu-based catalysts[2–5]describe their activity,selectivity and stability and show ways to improve them.The authors indicate changing catalyst composition—manipulating copper concentration or incorporating metal oxides(promoters)into Cu precursor as well as its subsequent activation to obtain the optimal catalyst.In our previous works,the influence of various promoters on the catalytic performance of CuZnZr catalyst in methanol steam reforming and methanol synthesis from carbon dioxide and hydrogen processes were investigated[6–8].The activity of the catalysts was evaluated in terms of the values of product yield and selectivity.The significance of selectivity is a consequence of the sensitivity of low temperature fuel cell electrodes to poisoning by several contaminants,especially CO.Particularly,parts of low temperature fuel cells such as proton exchange membranes(PEM)for small-scale applications impose high purity requirements for the hydrogen used.The stability of the studied catalysts was also taken into consideration.The best of the studied Ga promoted Cu/Zn/Zr catalysts exhibited high performance in the methanol steam reforming process.Papers on the influence of reduction conditions are very scarce.The only paper connected with that subject written by Idem et al.[2]described two activation methods—the reduction of the Cu catalyst with H2–N2atmosphere and with1:1M mixture of methanol and water.The catalyst activity in the process of methanol steam reforming was evaluated in terms of methanol conversion.However,the authors did not consider the selectivity of the catalyst.They found that catalysts activated by methanol–water feed provided higher activity(maximum methanol conversion degree increased about36%).In their investigation of CuO/ZnO catalysts in the methanol decomposition process,Xi et al.[9]showed that the activity of catalyst reduced with hydrogen was much higher than the activity of the ones reduced with methanol and carbon monoxide mixture.Fujita et al.[10]performed more detailed studies of the effects of the reduction conditions on the catalytic performance of Cu/ZnO catalyst for the methanol synthesis from CO2and H2.In their investigations,they applied several activation methods—the reduction catalyst with4%H2–He for three different temperature procedures,4%CH3OH,4%H2O–He,4%CH3OH–He.The highest Cu dispersion, activity and selectivity for the methanol formation was obtained for the catalyst reduced with methanol.They obtained even more than75%increase in the methanol production.In this work,the effects of the catalyst activation method of Cu/Zn/Zr/Ga catalyst are studied using hydrogen,carbon monoxide,pure methanol and1:1M mixture of methanol and water diluted in the stream of nitrogen as reductants. ExperimentalThe copper/zinc/zirconia/gallium catalyst used in these experiments was prepared in our laboratory using the thermal decomposition of citrates.Decomposing the citrate complexes of metals was performed according to the method described by Courty 123Steam reforming of methanol over Cu/Zn/Zr/Ga catalyst87 et al.[11].The required amounts of nitrates of Cu,Zn,Zr and Ga were added in small portions under intense stirring to a2M solution of citric acid(CA).The amount of CA used was calculated as1/3mol CA/g eq.of each metal plus extra 2%.The solution obtained,being an ideal mixture of all components,was evaporated in a rotatingflask in vacuum at363K until solid residue was obtained, then dried at403K for8h,then calcined on air for1h subsequently at373,473, 523and573K.The resultant powder was palletized,crushed and sized to obtain graining of1.2–2mm.The specific surface area of the oxide precursor and the catalyst after the reaction was measured with the BET method using an Autosorb-1Quantachrome apparatus with nitrogen as adsorbate at77.5K.The phase composition of the oxide precursor was determined with an XRD Siemiens D5005diffractometer.The grain size of the phases was calculated from the Debye–Scherrer formula.The active surface of copper in the reduced catalyst was determined with the use of reactive adsorption of N2O at363K.The catalyst(about0.5g)was reduced at523K for3h and cooled to363K.Then100l L of N2O pulses were injected until the reaction was completed.The amount of the reacted N2O was determined using a mass spectrometer(VG/Fisons Quartz200D).Activation methodsThe reductants and the procedures for catalyst reduction are listed below:1.10%H2–N2(50mL/min),the temperature was raised from room temperature to453K at2K/min,then to473K at0.5K/min and kept for2h,this procedure was repeated the next day starting from423K.2.5%CO–N2,the sameflow and temperature procedure as in method1.3.5%CH3OH,5%H2O–N2,the sameflow and temperature procedure as inmethod1(methanol and water was injected by Gilson pump into the nitrogen stream and evaporated).4.5%CH3OH–N2,the sameflow and temperature procedure as in method1,methanol was injected as in method3.Catalyst performance testThe catalytic activity was measured using a tubular,fixed-bedflow reactor of volume8cm3,made of stainless steel.The bed consisted of2g of the catalyst placed between two layers of ceramic grains(porcelain).The catalyst was reduced by the studied method and activated in the mixture of reactants by raising the temperature by steps of30K between473and553K every2h.The catalytic activity in methanol steam reforming was determined under the following conditions:atmospheric pressure,temperature of473K,methanol and water molar ratio of1,liquid feed rate of1.2mL/h,nitrogenflow of6L/h.The temperature of 473K corresponds to the middle methanol conversion degree and hydrogen yields values.After leaving the reactor,the gases were analyzed chromatographically with Varian Star3800and Star3600chromatographs.123chowska AnalysisThe inlet and outlet gases were analyzed with an on-line gas chromatograph—Varian Star3800using Carbo Plot column and TCD detector,in series with a Supelcowax megabore column and aflame ionization detector(FID).Methanol was analyzed on a Supelcowax megabore column,using a FID and the rest of the reaction mixture such as carbon oxides,hydrogen,nitrogen and methane with a Carbo Plot column using a TCD detector.Methanizer,i.e.a special catalytic bed was used in combination with the FID in order to improve sensitivity of CO detection.According to the Varian-GC producer,the FID/Methanizer sensitivity is about20ppb.The presence of other byproducts(oxygenates or hydrocarbons)was verified on GSQ and Alumina columns using Varian Star3600.Results and discussionThe composition of the studied catalyst was65.3wt%CuO,26.3wt%ZnO, 4.5wt%ZrO2and3.9wt%Ga2O3.Figs.1and2present dependences of methanol conversion and hydrogen yield on the temperature and Fig.3presents dependences of selectivity and carbon dioxide concentration on the temperature for the CuZnZrGa catalyst activated with standard hydrogen reduction.123Steam reforming of methanol over Cu/Zn/Zr/Ga catalyst89Analysis of the results(Table1)of our investigations do not indicate a significant influence the activation method of the catalyst on hydrogen yields.However,it can be observed that the catalysts activated with the mixture of methanol and water and123T a b l e 1P h y s i c o c h e m i c a l c h a r a c t e r i z a t i o n a n d t h e v a l u e s o f m e t h a n o l c o n v e r s i o n d e g r e e ,h y d r o g e n y i e l d s a n d s e l e c t i v i t y a t 473K f o r t h e c a t a l y s t s a c t i v a t e d w i t h s t u d i e d m e t h o d sM e t h o d R e d u c t a n t A v e r a g e c r y s t a l l i t e s i z eC o p p e r s u r f a c e a r e a (m 2/g C u )C a t a l y s t s u r f a c e a r e a (m 2/g C a t )M e t h a n o l c o n v e r s i o n d e g r e e (%)H y d r o g e n y i e l d (L /(k g c a t h ))S e l e c t i v i t y a(%)y C O b (%)C u (n m )Z n O (n m )1H y d r o g e n 16.66.38.720.787.355998.10.672C a r b o n m o n o x i d e 17.16.19.130.089.955698.10.223M e t h a n o l 19.66.28.423.690.257399.60.094M e t h a n o l ?W a t e r 17.76.17.523.492.6583100aS e l e c t i v i t y w a s d e fin e d a s :S ¼F C O 2=ðF C O 2þF C O Þ•100(%)bC a r b o n m o n o x i d e c o n c e n t r a t i o n s w e r e c a l c u l a t e d w i t h o u t n i t r o g e n90chowska123Steam reforming of methanol over Cu/Zn/Zr/Ga catalyst91 the pure methanol show very good selectivity reaching almost100%.The values of selectivity for the catalysts activated with hydrogen(traditional method)and carbon monoxide are admittedly only a little lower,but in the case of fuel-cell applications, selectivity has a great significance.Even traces of CO([10ppm)in the reformed gas deteriorate a Pt electrode and the cell performance is lowered dramatically.The differences between values of hydrogen yields for the studied activation methods are also slight but indicate that methanol and water and pure methanol are favored as reducing agents.The influence of the reduction conditions for Cu based catalyst selectivity in methanol steam reforming process is rather meager,but it seems that even a small improvement in catalyst performance can be useful.ConclusionsThe best Cu based catalyst for the hydrogen production in the methanol steam reforming process was obtained by activation with the1:1M mixture of methanol and water because of the improvement of selectivity obtained catalyst. References1.Apanel G,Johnson E(2004)Fuel Cells Bull XI:12–172.Idem RO,Bakhshi NN(1995)Ind Eng Chem Res34:1548–15573.Wu G-S,Mao D-S,Lu G-Z(2009)Catal Lett130:177–1844.Huang G,Liaw B-J,Jhang C-J,Chen Y-Z(2009)Appl Catal A358:7–125.Frank B,Jentoft FC,Soerijanto H,Kro¨hnert J,Schlo¨gl R,Schoma¨cker R(2007)J Catal246:177–1926.Słoczyn´ski J,Grabowski J,Kozłowska A,Lachowska M,Skrzypek J(2004)Stud Surf Sci153:161–164chowska M(2004)In_z Chem Proc25:1243–12478.Słoczyn´ski J,Grabowski R,Olszewski P,Kozłowska A,Stoch J,Lachowska M,Skrzypek J(2006)Appl Catal310:127–1379.Xi J,Wang Z,Lu G(2002)Appl Catal A225:77–8610.Fujita S,Moribe S,Kanamori Y,Kakudate M,Takezawa N(2001)Appl Catal A207:121–12811.Courty Ph,Ajot H,Marcilly Ch,Delmon B(1973)Powder Technol7:21–38123。
基于天然气自热重整的SOFC系统性能分析
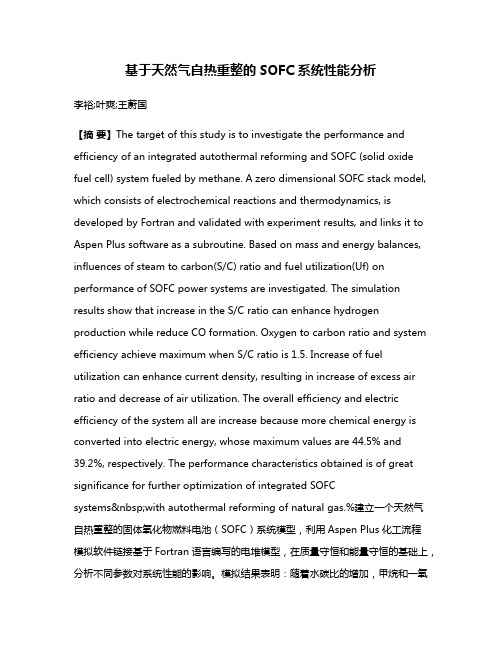
基于天然气自热重整的SOFC系统性能分析李裕;叶爽;王蔚国【摘要】The target of this study is to investigate the performance and efficiency of an integrated autothermal reforming and SOFC (solid oxide fuel cell) system fueled by methane. A zero dimensional SOFC stack model, which consists of electrochemical reactions and thermodynamics, is developed by Fortran and validated with experiment results, and links it to Aspen Plus software as a subroutine. Based on mass and energy balances, influences of steam to carbon(S/C) ratio and fuel utilization(Uf) on performance of SOFC power systems are investigated. The simulation results show that increase in the S/C ratio can enhance hydrogen production while reduce CO formation. Oxygen to carbon ratio and system efficiency achieve maximum when S/C ratio is 1.5. Increase of fuel utilization can enhance current density, resulting in increase of excess air ratio and decrease of air utilization. The overall efficiency and electric efficiency of the system all are increase because more chemical energy is converted into electric energy, whose maximum values are 44.5% and39.2%, respectively. The performance characteristics obtained is of great significance for further optimization of integrated SOFCsystems with autothermal reforming of natural gas.%建立一个天然气自热重整的固体氧化物燃料电池(SOFC)系统模型,利用Aspen Plus化工流程模拟软件链接基于Fortran语言编写的电堆模型,在质量守恒和能量守恒的基础上,分析不同参数对系统性能的影响。
Cu-ZrO2-CeO2
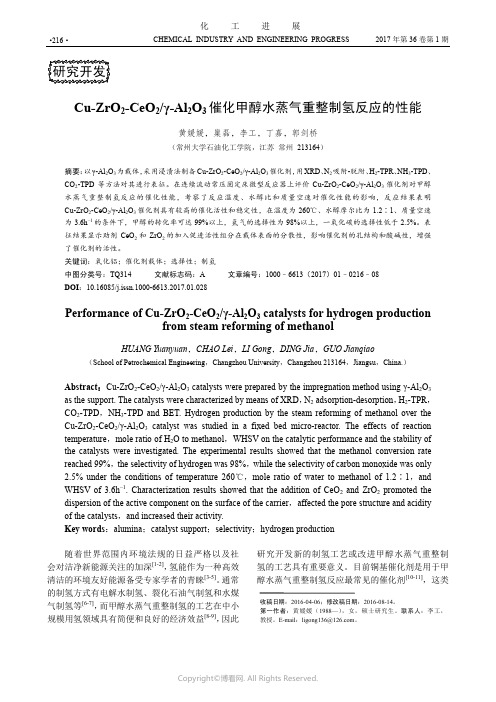
CHEMICAL INDUSTRY AND ENGINEERING PROGRESS 2017年第36卷第1期·216·化工进展Cu-ZrO2-CeO2/γ-Al2O3催化甲醇水蒸气重整制氢反应的性能黄媛媛,巢磊,李工,丁嘉,郭剑桥(常州大学石油化工学院,江苏常州 213164)摘要:以γ-Al2O3为载体,采用浸渍法制备Cu-ZrO2-CeO2/γ-Al2O3催化剂,用XRD、N2吸附-脱附、H2-TPR、NH3-TPD、CO2-TPD等方法对其进行表征。
在连续流动常压固定床微型反应器上评价Cu-ZrO2-CeO2/γ-Al2O3催化剂对甲醇水蒸气重整制氢反应的催化性能,考察了反应温度、水醇比和质量空速对催化性能的影响,反应结果表明Cu-ZrO2-CeO2/γ-Al2O3催化剂具有较高的催化活性和稳定性,在温度为260℃、水醇摩尔比为1.2∶1、质量空速为3.6h–1的条件下,甲醇的转化率可达99%以上,氢气的选择性为98%以上,一氧化碳的选择性低于2.5%。
表征结果显示助剂CeO2和ZrO2的加入促进活性组分在载体表面的分散性,影响催化剂的孔结构和酸碱性,增强了催化剂的活性。
关键词:氧化铝;催化剂载体;选择性;制氢中图分类号:TQ314 文献标志码:A 文章编号:1000–6613(2017)01–0216–08DOI:10.16085/j.issn.1000-6613.2017.01.028Performance of Cu-ZrO2-CeO2/γ-Al2O3 catalysts for hydrogen productionfrom steam reforming of methanolHUANG Yuanyuan,CHAO Lei,LI Gong,DING Jia,GUO Jianqiao(School of Petrochemical Engineering,Changzhou University,Changzhou 213164,Jiangsu,China.)Abstract:Cu-ZrO2-CeO2/γ-Al2O3 catalysts were prepared by the impregnation method using γ-Al2O3 as the support. The catalysts were characterized by means of XRD,N2 adsorption-desorption,H2-TPR,CO2-TPD,NH3-TPD and BET. Hydrogen production by the steam reforming of methanol over the Cu-ZrO2-CeO2/γ-Al2O3 catalyst was studied in a fixed bed micro-reactor. The effects of reaction temperature,mole ratio of H2O to methanol,WHSV on the catalytic performance and the stability of the catalysts were investigated. The experimental results showed that the methanol conversion rate reached 99%,the selectivity of hydrogen was 98%,while the selectivity of carbon monoxide was only2.5% under the conditions of temperature 260℃,mole ratio of water to methanol of 1.2∶1,andWHSV of 3.6h–1. Characterization results showed that the addition of CeO2 and ZrO2 promoted the dispersion of the active component on the surface of the carrier,affected the pore structure and acidity of the catalysts,and increased their activity.Key words:alumina;catalyst support;selectivity;hydrogen production随着世界范围内环境法规的日益严格以及社会对洁净新能源关注的加深[1-2],氢能作为一种高效清洁的环境友好能源备受专家学者的青睐[3-5]。
甲烷干重整研究进展
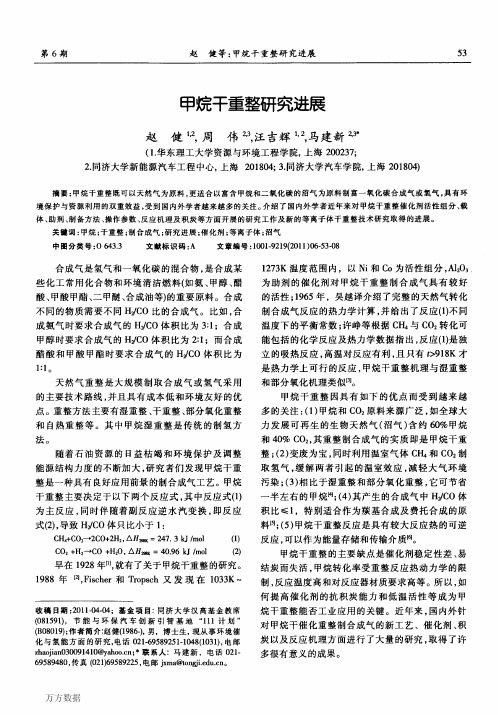
万方数据万方数据万方数据万方数据万方数据万方数据万方数据万方数据万方数据甲烷干重整研究进展作者:赵健, 周伟, 汪吉辉, 马建新, ZHAO Jian, ZHOU Wei, WA NG Ji-hui, MA Jian-xin作者单位:赵健,汪吉辉,ZHAO Jian,WA NG Ji-hui(华东理工大学资源与环境工程学院,上海200237;同济大学新能源汽车工程中心,上海201804), 周伟,马建新,ZHOU Wei,MA Jian-xin(同济大学新能源汽车工程中心,上海201804;同济大学汽车学院,上海201804)刊名:天然气化工英文刊名:Natural Gas Chemical Industry年,卷(期):2011,36(6)1.程金民;黄伟;左志军碳化终温对碳化钼的制备及甲烷二氧化碳重整催化性能的影响[期刊论文]-高等学校化学学报2010(01)2.Hanif A Study on the structure and formation mechanism of molybdenum carbides[外文期刊] 2002(03)3.Nagaoka K;Takanabe K;Aika K I Influence of the reduction temperature on catalytic activity of Co/TiO2 (anatase-type) for high pressure dry reforming of methane[外文期刊] 2003(01)4.Ghorbanzadeh A M;Lotfalipour R;Rezaei S Carbon dioxide reforming of methane at near room temperature in low energy pulsed plasma 20095.Zhang J;H Wang;A K Dalai Development of stable bimetallic catalysts for carbon dioxide reforming of methane [外文期刊] 2007(02)6.史克英;商永臣天然气二氧化碳转化制合成气的研究:Ⅸ.反应机理 1998(01)7.陈文艳环境友好条件下甲烷等离子体重整制氢的研究[学位论文] 20098.柴晓燕;尚书勇;刘改焕常压高频冷等离子体炬制备的CH4/CO2重整用Ni/Υ-Al2O3催化剂的表征[期刊论文]-催化学报2010(03)9.胡诗婧;龙华丽;徐艳冷等离子体喷射流对甲烷二氧化碳重整用Ni/Al2O3催化剂的还原机制[期刊论文]-催化学报 2011(02)10.郎宝;李金秀;季福生镧助剂对模拟生物沼气重整制备合成气中Ni/SBA-15催化剂结构和性能的影响[期刊论文]-物理化学学报 2009(08)11.Múnera J F Kinetics and reaction pathway of the CO2 reforming of methane on Rh supported on lanthanumbased solid[外文期刊] 2007(01)12.徐军科;周伟;汪吉辉Ni/La2O3/Al2O3催化剂上甲烷干重整积炭表征与分析[期刊论文]-催化学报 2009(11)13.Haghighi M;Sun Z Q;Wu J H On the reaction mechanism of CO2 reforming of methane over a bed of coal char[外文期刊] 2007(02)14.孙志强;吴晋沪;张东柯甲烷和二氧化碳在煤焦上反应制备合成气实验研究[期刊论文]-燃料化学学报 2009(06)15.Koo K Y Coke study on MgO-promoted Ni/Al2O3 catalyst in combined H2O and CO2 reforming of methane for gasto liquid (GTL) process[外文期刊] 2008(02)16.Yah B H;Wang Q;Jin Y Dry reforming of methane with carbon dioxide using pulsed DC arc plasma at atmospheric pressure 201017.Nandini A;Pant K K;Dhingra S C Kinetic study of the catalytic carbon dioxide reforming of methane to synthesis gas over Ni-K/CeO2-Al2O3 catalyst[外文期刊] 2006(0)18.Alstrup I M;Tavares T The kinetics of carbon formation from CH4 +H2 on a silica-supported nickel catalyst [外文期刊] 1992(01)19.Solymosi F;Erd helyi A;Cserényi J A comparative study on the activation and reactions of CH4 on supported metals[外文期刊] 1992(04)20.Erdohelyi A;Cserenyi J;Solymosi F Activation of CH4 and its reaction with CO2 over supported Rh catalysts [外文期刊] 1993(01)21.Solymosi F The bonding,structure and reactions of CO2 adsorbed on clean and promoted metal surfaces[外文期刊] 1991(03)22.Tao X m;Qi F w;Yin Y X CO2 reforming of CH4 by combination of thermal plasma and catalyst[外文期刊]2008(04)23.Goujard V;Tatibou(e)t J M;Batiot-Dupeyrat C Influence of the plasma power supply nature on the plasmacatalyst synergism for the carbon dioxide reforming of methane[外文期刊] 2009(12)24.Xiao T Effect of carburising agent on the structure of molybdenum carbides[外文期刊] 2001(12)25.Claridge J Study of the temperature-programmed reaction synthesis of early transition metal carbide and nitride catalyst materials from oxide precursors[外文期刊] 2000(01)26.Tao X M;Bai M G;Wu Q Y CO2 reforming of CH4 by binode thermal plasma[外文期刊] 200927.Pritchard M The effects of sulfur and oxygen on the catalytic activity of molybdenum carbide during dry methane reforming[外文期刊] 2004(1-2)28.Claridge J New catalysts for the conversion of methane to synthesis gas:Molybdenum and tungsten carbide[外文期刊] 1998(01)29.York A P E Synthesis of early transition metal carbides and their application for the reforming of methane to synthesis gas 199730.王芳;归柯庭CH4-CO2的高温半焦重整反应实验[期刊论文]-东南大学学报(自然科学版) 2008(01)31.柳海涛;田宏;王晓来Mo、W金属氧化物对CH4/CO2重整Ni基催化剂性能的影响[期刊论文]-分子催化 2007(04)32.Valderrama G;Kiennemann A;Goldwasser M R Dry reforming of CH4 over solid solutions of LaNi1-xCoxO333.Araujo G C Catalytic evaluation of perovskite-type oxide LaNi1-xRuxO3 in methane dry reforming34.Rezaei M CO2 reforming of CH4 over nanocrystalline zirconia-supported nickel catalysts[外文期刊] 2008(3-4)35.Damyanova S;Bueno J M C Effect of CeO2 loading on the surface and catalytic behaviors of CeO2-Al2O3-supported Pt catalysts 2003(01)36.钱岭;阎子峰担载型镍基催化剂上甲烷二氧化碳重整反应机理的研究[期刊论文]-复旦学报(自然科学版) 2003(03)37.Gallego G S CO2 reforming of CH4 over La-Ni based perovskite precursors[外文期刊] 2006(0)38.Zhang J;Wang H;Dalai A K Effects of metal content on activity and stability of Ni-Co bimetallic catalysts for CO2 reforming of CH4[外文期刊] 2008(02)39.董跃;张勇发;张国杰CH4-CO2重整反应过程中炭催化剂失重特性[期刊论文]-化学反应工程与工艺 2009(01)40.Shamsi A;C D Johnson Effect of pressure on the carbon deposition route in CO2 reforming of 13CH4[外文期刊] 2003(1-2)41.陈吉祥;王日杰;张继炎压力对CH4/CO2重整制取合成气反应性能的影响[期刊论文]-化学反应工程与工艺 2005(04)42.王玉和;徐柏庆加压下Ni/MgO催化剂催化CO2重整CH4反应的特性[期刊论文]-催化学报 2005(04)43.赵金保;J R H Ross CH4-CO2高压反应中水蒸气对催化剂稳定性的影响 1998(06)44.张丙模;张永发高压CH4-CO2重整制合成气研究进展[期刊论文]-山西能源与节能 2009(05)45.Gross B;Crycz B;MIklossy K等离子体技术 198046.郭芳;储伟;石新雨等离子体引入方式对强化制备二氧化碳重整甲烷反应的Ni/Υ-Al2O3催化剂的影响[期刊论文]-高等学校化学学报 2009(04)47.Hua W Preparation of Ni/MgO catalyst for CO2 reforming of methane by dielectric-barrier discharge plasma[外文期刊] 2010(11)48.Shang S Y;Liu G H;Chai X Y Research on Ni/Υ-Al2O3 catalyst for CO2 reforming of CH4 prepared by atmospheric pressure glow discharge plasma jet[外文期刊] 200949.Liu G H;Chu W;Long H L A novel reduction method for Ni/Υ-Al2O3 catalyst by a high frequency cold plasma jet at atmospheric pressure[期刊论文]-Chinese Journal of Catalysis 2007(07)50.Pan Y X;Liu C J;Cui L Temperature-programmed studies of coke resistant Ni catalyst for carbon dioxide reforming of methane[外文期刊] 2008(01)51.Hao Z Characterization of aerogel Ni/Al2O3 catalysts and investigation on their stability for CH4-CO2 reforming in a fluidized bed[外文期刊] 2009(01)52.Wang N Effects of Ce/Zr ratio on the structure and performances of Co-Ce1-xZrxO2 catalysts for carbon dioxide reforming of methane[期刊论文]-Journal of Natural Gas Chemistry 2010(02)53.Kambolis A Ni/CeO2-ZrO2 catalysts for the dry reforming of methane[外文期刊] 2010(1-2)54.余长春;丁雪加CO2与CH4催化反应合成气研究 1993(02)55.Daza C E Syngas production from CO2 reforming of methane using Ce-doped Ni-catalysts obtained from hydrotalcites by reconstruction method[外文期刊] 2010(02)56.Ruiz J A C Syngas production by autothermal reforming of methane on supported platinum catalysts[外文期刊] 2008(1-2)57.李兆静;周伟;徐军科La2O3对Ni/α-Al2O3催化剂结构及沼气重整制氢性能的影响[期刊论文]-天然气化工 2009(04)58.徐军科;汪吉辉;李兆静La2O3改进Ni/Υ-Al2O3催化剂上沼气重整制氢[期刊论文]-太阳能学报 2009(05)59.Zhang S One-pot synthesis of Ni-nanoparticle-embedded mesoporous titania/silica catalyst and itsapplication for CO2-reforming of methane[外文期刊] 2008(06)60.胡全红;黎先财;杨爱军Ni/BaTiO3/Υ-Al2O3催化剂的结构及其甲烷二氧化碳重整性能研究[期刊论文]-天然气化工2009(03)61.王建坤甲烷催化重整制合成气反应的Ni基复合催化剂的研究[学位论文] 200962.Tao X M;Bai M G;Li X CH4-CO2 reforming by plasma-challenges and opportunities 2010(22)63.Tao K Development of platinum-based bimodal pore catalyst for CO2 reforming of CH4[外文期刊] 2010(3-4)64.Pinheiro A N Highly stable dealuminated zeolite support for the production of hydrogen by dry reforming of methane[外文期刊] 2009(1-2)65.李延兵;肖睿;金保升焦炭体系下二氧化碳重整甲烷制取合成气[期刊论文]-燃烧科学与技术 2009(03)66.李玉洁;王鹏;赵炜半焦对富含甲烷气体转化制备合成气的作用(Ⅱ):改性半焦对CO和H2反应生成CH4的作用[期刊论文]-煤炭转化 2007(01)67.Suelves I;Lázaro M J;Moliner R Hydrogen production by methane decarbonization:Carbonaceous catalysts[外文期刊] 2007(15)68.陈娟荣;黎先财;杨沂凤BaTiO3负载Ni-Co双金属催化剂催化CH4/CO2重整反应[期刊论文]-天然气化工 2007(04)69.陈俭省;李凝;刘金聚掺杂氧化物对Ni-ZrO2-Al2O3催化剂的性能影响[期刊论文]-广西科学 2010(01)70.徐文嫒;龙威;杜瑞焕CH4-CO2重整反应中工业技术的运用[期刊论文]-化工时刊 2010(01)71.Pawelec B;Damyanova S;Arishtirova K Structural and surface features of PtNi catalysts for reforming of methane with CO2[外文期刊] 2007(0)72.Wang Q;Cheng Y;Jin Y Dry reforming of methane in an atmospheric pressure plasma fluidized bed with Ni/Υ-Al2O3 catalyst 200973.Zhang A J;Zhu A M;Guo J Conversion of greenhouse gases into syngas via combined effects of discharge activation and catalysis 201074.Long H L;Shang S Y;Tao X M CO2 reforming of CH4 by combination of cold plasma jet and Ni/Υ-Al2O3 catalyst [外文期刊] 2008(20)75.Bo Z;Yan J;Li X Plasma assisted dry methane reforming using gliding arc gas discharge:effect of feed gases proportion 2009(20)76.Chun Y N;Yang Y C;Yoshikawa K Hydrogen generation from biogas reforming using a gliding arc plasma-catalyst reformer[外文期刊] 2009(3-4)77.Yang Y C;Lee B J;Chun Y N Characteristics of methane reforming using gliding arc reactor[外文期刊] 2009(2)78.郝世雄;余祖孝;刘兴勇甲烷二氧化碳催化重整制合成气研究进展[期刊论文]-化学世界 2010(05)79.Rico V J;Hueso J L;Cotrino J Evaluation of different dielectric barrier discharge plasma configurations as an alternative technology for green Cl chemistry in the carbon dioxide reforming of methane and the direct decomposition of methanol[外文期刊] 201080.Wang Q;Yah B H;Jin Y Investigation of dry reforming of methane in a dielectric barrier discharge reactor[外文期刊] 2009(3)81.黄珍介质阻挡放电条件下甲烷二氧化碳重整制备合成气的研究[学位论文] 200882.朱威娜非平衡等离子体用于甲烷二氧化碳重整制合成气的研究[学位论文] 200883.Hwang N;Song Y H;Cha M S Efficient use of CO2 reforming of methane with an arc-jet plasma[外文期刊] 201084.Li D H;Li X;Bai M G CO2 reforming of CH4 by atmospheric pressure glow discharge plasma:A high conversion ability 200985.Li X;Bai M G;Tao X M Carbon dioxide reforming of methane to synthesis gas by an atmospheric pressure plasma jet[期刊论文]-Journal of Fuel Chemistry and Technology 2010(02)86.Li X;Tao X M;Yin Y X An atmospheric-pressure glowdischarge plasma jet and its application 2009(06)87.李祥;白玫瑰;陶旭梅大气压等离子体射流重整CH4-CO2制合成气[期刊论文]-燃料化学学报 2010(02)88.钟犁;严建华;薄拯滑动弧放电等离子体重整甲烷制取合成气[期刊论文]-浙江大学学报(工学版) 2010(03)89.Bradford M C J;Vannice M A CO2 reforming of CH4[外文期刊] 1999(01)1.徐军科.任克威.王晓蕾.周伟.潘相敏.马建新.XU Jun-ke.REN Ke-wei.WANG Xiao-lei.ZHOU Wei.PAN Xiang-min.MA Jian-xin甲烷干重整制氢研究进展[期刊论文]-天然气化工2008,33(6)2.徐海燕.雷廷宙.任素霞.何晓峰.朱金陵.Xu Haiyan.Lei Tingzhou.Ren Suxia.He Xiaofeng.Zhu Jinling生物质合成气一步法合成二甲醚CuZnFeZr/HZSM-5催化剂的研究[期刊论文]-河南科学2012,30(2)3.Takaku Yamamoto.Hirotaka Sato.Yoshinori Matsukura.Yutaka Ujisawa利用钢铁生产吹氧法的垃圾气化与熔融系统[期刊论文]-钢铁2003,38(z1)4.丁荣刚.闫子峰.宋林花.刘欣梅.Ding Ronggang.YAN Zifeng.Song Linhua.LIU Xinmei甲烷干气重整反应进展[期刊论文]-天然气化学(英文版)2001,10(3)5.王铁军.常杰.吕鹏梅.张喜通.鲁皓沼气重整生物质富氢燃气合成醇醚燃料[会议论文]-20046.汪家铭干重整技术利用甲烷和二氧化碳制合成气[期刊论文]-大氮肥2011,34(4)7.王铁军.常杰.吕鹏梅.祝京旭生物质间接液化一步法合成燃料二甲醚[期刊论文]-煤炭转化2003,26(4)8.薛冰.冯杰.李文英双气头多联产中醇醚燃料合成流程的经济评价[会议论文]-20099.刘晓凤.刘莉.尹小波.徐恒.张辉.邓宇.胡国全.张敏.LIU Xiao-feng.LIU Li.YIN Xiao-bo.XU Heng.ZHANG Hui.DENG Yu. HU Guo-quan.ZHANG Ming一氧化碳的生物甲烷化研究进展[期刊论文]-中国沼气2005,23(4)10.郭秀兰.赵月春.黄鹤生物质催化气化合成甲醇[期刊论文]-能源工程2004(1)本文链接:/Periodical_trqhg201106012.aspx。
微型反应器中生物质甲醇催化转化制氢的研究

微型反应器中生物质甲醇催化转化制氢的研究马克东;穆昕;周毅;毕怡;张磊;潘立卫【摘要】笔者创新研制了一种多层板式微型制氢反应器,集甲醇催化燃烧、重整和原料预热于一体;通过计算物流速度分布,合理地设计了单板结构和几何尺寸;反应器依靠液体甲醇的催化燃烧实现水蒸汽重整制氢过程自热运行.考察了反应器启动、变载过程以及稳态性能和寿命,结果表明,当温度为320℃,空速为1600 h-1,水醇比为1.2时,甲醇转化率为100%,重整气中H2 74.46%,CO2 24.17%,C0 1.37%;重整腔甲醇空速为1350~1600 h-1,燃烧腔进料量为每min 0.158 mL,反应器可连续运行60 h,甲醇转化率在98%以上;反应器能量效率最高为45%,最大产氢量接近10.74 L·h-1.【期刊名称】《中国沼气》【年(卷),期】2016(034)002【总页数】4页(P9-12)【关键词】微型反应器;制氢;催化转化;甲醇水蒸汽重整;氢源【作者】马克东;穆昕;周毅;毕怡;张磊;潘立卫【作者单位】大连大学环境与化学工程学院,辽宁大连116622;中国科学院大连化学物理研究所,辽宁大连116023;大连大学环境与化学工程学院,辽宁大连116622;大连大学环境与化学工程学院,辽宁大连116622;辽宁石油化工大学化学化工与环境学部,辽宁抚顺113001;大连大学环境与化学工程学院,辽宁大连116622;中国科学院大连化学物理研究所,辽宁大连116023【正文语种】中文【中图分类】S216.4;TK6氢能以其清洁来源及用途广泛等优点成为最有希望的替代能源之一,用可再生能源制氢是氢能发展的必然趋势[1-3]。
基于生物质的甲烷、甲醇、乙醇的化学重整转化制氢由于其独特的优点,已成为发展氢经济颇具前景的研究领域之一[4-6]。
在我国,氢能研究的前期工作主要是围绕燃料电池汽车展开[7],由于成本高以及基础设施投入庞大,短时间内尚无法进入市场实际应用。
005NGC部分氧化
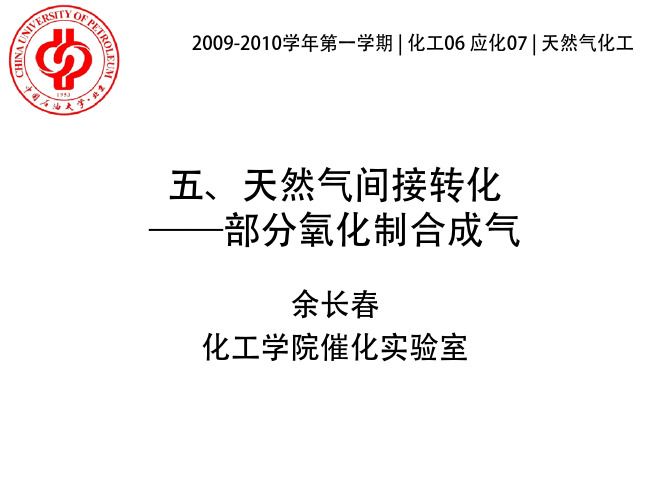
五、天然气间接转化 ——部分氧化制合成气
余长春 化工学院催化实验室
/ 化工06 / 应化07 / 天然气化工
部分氧化法造气 - 基本化学反应
部分氧化法是将烃(煤、生物质)与氧在高温下转化为氢气和一氧 化碳的方法。可以单独使用,大多数是与其他过程联合使用
/ 化工06 / 应化07 / 天然气化工
联合转化特点
在转化和合成气压缩这两个步骤上节省了能 量, 也提高了能量利用效率。与常规蒸汽转 化相比
每吨甲醇的天然气耗量将减少8%~10% 所增加的空分装置投资和压缩氧气所增加的成 本, 均可由操作费用的节约而得到弥补
/ 化工06 / 应化07 / 天然气化工
/ 化工06 / 应化07 / 天然气化工
GHR流程示意图
/ 化工06 / 应化07 / 天然气化工
ATR工艺
Topsoe公司开发的ATR工艺不仅把蒸汽转化和部分氧化结 合在同一个反应器中进行, 同时还取消了蒸汽转化用的炉管 ATR工艺反应器上部为燃烧段, 在此使一定量的甲烷进行部 分氧化反应, 总氧量和烃类的摩尔比为 0.55~0.60 由于一级氧化产物CO再氧化为CO2的速度较慢, 故部分氧化 反应有很高的选择性 燃烧段下面是固定床蒸汽催化转化反应段, 利用燃烧段释放 的热量进行蒸汽转化反应 Topsoe公司已在天然气处理量为 100m3/h的装置上完成了 中间试验, 其结果如下页表所示
/ 化工06 / 应化07 / 天然气化工
针对不同用途的合成气制造流程
NG, natural gas HDS, desulfurization, TSR, tubular steam reforming SR, secondary reforming WGS, water gas shift, Meth, methanation PSA, pressure swing adsorption PR, pre-reforming GTL, gas-to-liquid ATR, autothermal reforming FT, Fischer–Tropsch synthesis HC, hydrocracking
天然气制乙烯技术进展及经济性分析

2016年第35卷第6期CHEMICAL INDUSTRY AND ENGINEERING PROGRESS ·1733·化工进展天然气制乙烯技术进展及经济性分析胡徐腾(中国化工集团公司,北京 100080)摘要:介绍了当前几种主要的天然气制乙烯技术新进展,包括天然气经甲醇制乙烯、费-托合成制乙烯、甲烷氧化偶联制乙烯技术进展及应用情况,并对这几种工艺进行了技术经济评价,结论认为:天然气制乙烯技术的大规模应用,主要取决于天然气原料供应的有效保障及其价格是否合理,在天然气供应充足、价格合理的条件下,天然气经甲醇制乙烯工艺将会得到较快发展,而费-托合成制乙烯、甲烷氧化偶联制乙烯技术目前尚未达到成熟应用阶段,需要持续加大研发力度,争取早日实现工业化应用。
关键词:天然气;乙烯;甲醇;费-托合成;甲烷氧化偶联;技术进展;经济评价中图分类号:TQ 221.21 文献标志码:A 文章编号:1000–6613(2016)06–1733–06DOI:10.16085/j.issn.1000-6613.2016.06.013Technology progress and economy analysis on natural gas to ethyleneHU Xuteng(China National Chemical Corporation,Beijng 100080,China)Abstract:The current main technology progress of natural gas to ethylene were introduced,including natural gas to ethylene through methanol,Fischer-Tropsch synthesis route,and oxidative coupling of methane. Technical and economic evaluation of these processes were performed,and the conclusions are:the large-scale application of natural gas to ethylene technology mainly depends on the natural gas supply and it’s price. Under the conditions of sufficient gas supply and reasonable price,the technology of natural gas to ethylene through methanol will get a rapid development,while the applications of Fischer-Tropsch synthesis and oxidative coupling of methane technology at present has not yet been mature and it is necessary to continue to intensify their research and development for the realization of their industrial application.Key words: natural gas;ethylene;methanol;Fischer-Tropsch synthesis; oxidative coupling of methane;technical progress;economic evaluation近年来,全球乙烯市场需求强劲,2014年全球乙烯产能达到1.53亿吨/年,需求量1.43亿吨,产量1.3亿吨,由于供应增速低于需求增速,市场供应相对偏紧[1]。
托普索公司介绍
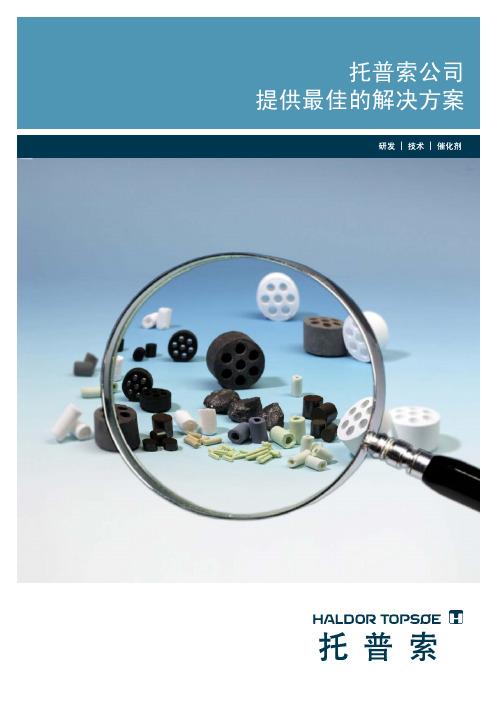
研发 | 技术 | 催化剂
“只有坚持研发,我们才能不断向客户提供最好的产品。”
— Jesper Nerlov 托普索公司执行副总裁
托普索研发中心的世界上最先进的电子显微镜 用于原子级催化领域研究
专注于研发
从公司成立之初,托普索博士就坚持一个理念,即理想的业务 发展模式是基于不断的产品研发和新市场的开拓。由于一直秉 承这一理念,我们能够向客户提供最先进、最可靠,以及最有 经济性的产品。
工艺责任—一揽子产品和服务
托普索能够为客户提供从催化剂到工艺包的一揽子产品和服 务,这样不仅方便了客户,节约了时间和成本,更明晰了工艺 责任。托普索的供货范围包括:
- 基础工艺设计 - 关键设备和工艺的详细设计 - 催化剂供货 - 专有设备供货 - 采购和监造服务 - 装置操作人员的培训 - 装置施工期间的指导服务 - 调试和开车期间的协助 - 装置运行期间的技术服务和运行优化服务
托普索的社会责任
能源、环境和粮食是当今世界人们最关心的问题。而托普索的 催化剂和技术正在这些领域中起着重要作用。我们对托普索的 产品感到无比骄傲,因为它们的确为工艺节能、为环境,以及 为全球能源的使用方式带来了实实在在的变化。
位于丹麦哥本哈根北部Lynby的托普索总部
托普索里程碑
1940年 1944年 1957年 1958年
柴油自热重整制氢催化剂及其活性分析
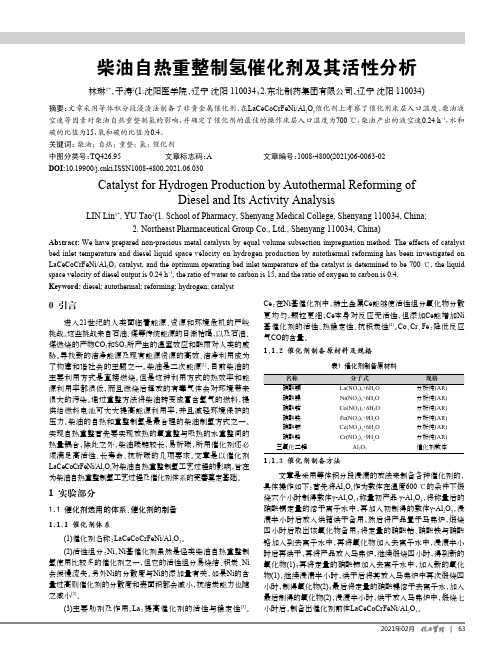
Ce :在Ni 基催化剂中,稀土金属Ce 能够使活性组分氧化物分散更均匀,颗粒更细,Ce 本身对反应无活性,但添加Ce 能增加Ni 基催化剂的活性、热稳定性、抗积炭性[4]。
Co 、Cr 、Fe :降低反应气CO 的含量。
1.1.2 催化剂制备原材料及规格表1 催化剂制备原材料332硝酸镍Ni(NO 3)2·6H 2O 分析纯(AR)硝酸钴Co(NO 3)2·6H 2O 分析纯(AR)硝酸铁Fe(NO 3)3·9H 2O 分析纯(AR)硝酸铈Ce(NO 3)3·6H 2O 分析纯(AR)硝酸铬Cr(NO 3)3·9H 2O 分析纯(AR)三氧化二铝Al 2O 3催化剂载体1.1.3 催化剂制备方法文章是采用等体积分段浸渍的放法来制备各种催化剂的,具体操作如下:首先将Al 2O 3作为载体在温度600 ℃的条件下煅烧六个小时制得载体γ-Al 2O 3;称量初产品 γ-Al 2O 3,将称量后的硝酸镧定量的溶于离子水中,再加入初制得的载体γ-Al 2O 3,浸渍半小时后放入烘箱烘干备用,然后将产品置于马弗炉,煅烧四小时后取出该氧化物备用;将定量的硝酸钴、硝酸铁与硝酸铬加入到去离子水中,再将氧化物加入去离子水中,浸渍半小时后再烘干,再将产品放入马弗炉,继续煅烧四小时,得到新的氧化物(1);再将定量的硝酸铈加入去离子水中,加入新的氧化物(1),继续浸渍半小时,烘干后将其放入马弗炉中再次煅烧四小时,制得氧化物(2);最后将定量的硝酸镍溶于去离子水,加入最后制得的氧化物(2),浸渍半小时,烘干放入马弗炉中,煅烧七小时后,制备出催化剂前体LaCeCoCrFeNi/Al 2O 3。
0 引言进入21世纪的人类面临着能源、资源和环境危机的严峻挑战。
这些挑战来自石油、煤等传统能源的日渐枯竭,以及石油、煤燃烧的产物CO 2和SO 2所产生的温室效应和酸雨对人类的威胁。
寻找新的洁净能源及现有能源资源的高效、洁净利用成为了构建和谐社会的主题之一。
Fischer-Tropsch process
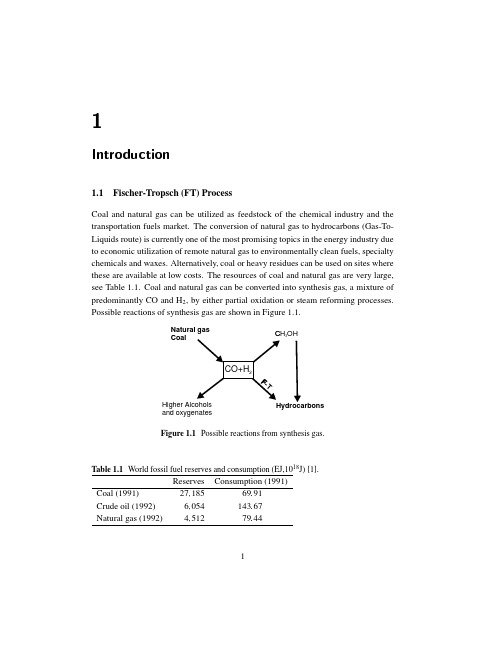
1.1Fischer-Tropsch(FT)ProcessCoal and natural gas can be utilized as feedstock of the chemical industry and the transportation fuels market.The conversion of natural gas to hydrocarbons(Gas-To-Liquids route)is currently one of the most promising topics in the energy industry due to economic utilization of remote natural gas to environmentally clean fuels,specialty chemicals and waxes.Alternatively,coal or heavy residues can be used on sites where these are available at low costs.The resources of coal and natural gas are very large, see Table1.1.Coal and natural gas can be converted into synthesis gas,a mixture of predominantly CO and H2,by either partial oxidation or steam reforming processes. Possible reactions of synthesis gas are shown in Figure1.1.Natural gasCoalFigure1.1Possible reactions from synthesis gas.Table1.1World fossil fuel reserves and consumption(EJ,1018J)[1].Coal(1991)271856991Crude oil(1992)605414367Natural gas(1992)451279442C HAPTER1Table1.2Major overall reactions in the Fischer-Tropsch synthesis.Side reactions4.Alcohols2n H2+n CO C n H2n2O+n1H2O5.Boudouard reaction2CO C+CO2The conversion of the synthesis gas to aliphatic hydrocarbons over metal catalysts was discovered by Franz Fischer and Hans Tropsch at the Kaiser Wilhelm Institute for Coal Research in M¨u llheim in1923[2,3].They proved that CO hydrogenation over iron,cobalt or nickel catalysts at180-250˚C and atmospheric pressure results in a product mixture of linear hydrocarbons.The Fischer-Tropsch product spectrum con-sists of a complex multicomponent mixture of linear and branched hydrocarbons and oxygenated products.Main products are linear paraffins and-olefins.The overall reactions of the Fischer-Tropsch synthesis are summarized in Table1.2.The hydro-carbon synthesis is catalyzed by metals such as cobalt,iron,and ruthenium.Both iron and cobalt are used commercially these days at a temperature of200to300˚C and at 10to60bar pressure[4,5].The reactions of the FT synthesis on iron catalysts can be simplified as a combi-nation of the FT reaction and the water gas shift(WGS)reaction:CO1m2n H21n C n H m H2O F T-H FT=165kJ/mol(1.1) CO H2O CO2H2W GS-H W GS=41.3kJ/mol(1.2)where n is the average carbon number and m is the average number of hydrogen atoms of the hydrocarbon products.Water is a primary product of the FT reaction,and CO2 can be produced by the WGS reaction.The WGS activity can be high over potassium-promoted iron catalysts and is negligible over cobalt or ruthenium catalysts.I NTRODUCTION3Figure1.2shows a block diagram of the overall Fischer-Tropsch process config-uration.The commercial process involves three main sections,namely:synthesis gas production and purification,Fischer-Tropsch synthesis,and product grade-up.These subjects are described in more detail below.Choi et al.[6]gives a capital cost break-down of these three individual process sections for a45,000bbl/day FT plant.The synthesis gas preparation(that is air separation plant,partial oxidation,steam reform-ing of natural gas,and syngas cooling)is about66%of the total on-site capital costs. The FT synthesis section consisting of FT slurry reactors,CO2removal,synthesis gas compression and recycle,and recovery of hydrogen and hydrocarbons is22%of the total costs.Finally,the upgrading and refining section of hydrocarbons is about12 %.Consequently,cost reduction of synthesis gas production is the most beneficial. Note,however,that at afixed production rate the selectivity of the FT process directly affects the size of the syngas generation section.A high selectivity of the FT process to desired products is of utmost importance to the overall economics.1.1.1Synthesis Gas ProductionSynthesis gas can be obtained by steam reforming or(catalytic)partial oxidation of fossil fuels:coal,natural gas,refinery residues,biomass or industrial off-gases.The composition of syngas from the various feedstocks and processes is given in Table1.3 [7,8].Synthesis gas can be obtained from reforming of natural gas with either steam or carbon dioxide,or by partial oxidation.The most important reactions are:Steam reforming CH4+H2O CO+3H2CO2reforming CH4+CO22CO+2H2Partial oxidation CH4+14C HAPTER1Figure1.2Overall process scheme Fischer-Tropsch.I NTRODUCTION5 Table1.3Synthesis gas compositions.Natural gas,steam SR173.815.56641 Natural gas,steam,CO2CO2-SR252.326.185131 Natural gas,O2,ATR260.230.27520 steam,CO2Coal/heavy oil,steam Gasification167.828.72906 Coal,steam,oxygen Texaco gasifier135.151.810625 Coal,steam,oxygen Shell/Koppers gasifier130.166.12513 Coal,steam,oxygen Lurgi gasifier339.118.92971236C HAPTER 10.4NaturalFigure 1.3Feedstocks and catalysts [10].for development of commercial Fischer-Tropsch reactors are the high reaction heats and the large number of products with varying vapor pressures (gas,liquid,and solid hydrocarbons).The main reactor types which have been proposed and developed after 1950are [5,15,16]:1.Three-phase fluidized (ebulliating)bed reactors or slurry bubble column reac-tors with internal cooling tubes (SSPD:Sasol;GasCat:Energy International,AGC-21:Exxon,see Figure 1.4a)2.Multitubular fixed bed reactor with internal cooling (Arge:Sasol;SMDS:Shell,see Figure 1.4b)3.Circulating fluidized bed reactor with circulating solids,gas recycle and cooling in the gas/solid recirculation loop (Synthol:Sasol)(Figure 1.4c)4.Fluidized bed reactors with internal cooling (SAS:Sasol)(Figure 1.4d)Sie [5]compared the advantages and disadvantages of the two most favorite reactor systems for the Fischer-Tropsch synthesis of high molecular weight products:that is the multitubular trickle bed reactor and the slurry bubble column reactor.Major drawbacks of the bubble column are requirements for continuous separation betweenI NTRODUCTION7 coolant8C HAPTER11.1.3Product Upgrading and SeparationConventional refinery processes can be used for upgrading of Fischer-Tropsch liquid and wax products.A number of possible processes for FT products are:wax hydro-cracking,distillate hydrotreating,catalytic reforming,naphta hydrotreating,alkylation and isomerization[6,20].Fuels produced with the FT synthesis are of a high quality due to a very low aromaticity and zero sulfur content.The product stream consists of various fuel types:LPG,gasoline,diesel fuel,jet fuel.The definitions and conventions for the composition and names of the different fuel types are obtained from crude oil refinery processes and are given in Table1.4.Table1.4Conventions of fuel names and composition[1].Fuel gas C1-C2LPG C3-C4Gasoline C5-C12Naphtha C8-C12Kerosene Jet fuel C11-C13Diesel Fuel oil C13-C17Middle distillates Light gas oil C10-C20Soft wax C19-C23Medium wax C24-C35Hard wax C35I NTRODUCTION9 Table1.5Product quality,adapted from Sie[5]and Gregor[22].Diesel Cetane number7074min.40Cloud point,˚C-10-7-20to+201.2Industrial Fischer-Tropsch ProcessesBelow,the major industrial Fischer-Tropsch processes are discussed briefly.The em-phasis is on processes developed after1980.Table1.6gives an overview of the major companies and their patents divided in the following sections:1.FT catalyst devel-opment;2.process design and development;3.upgrading of specific FT products.A comparison of the several industrial Fischer-Tropsch companies is presented in Ta-ble1.7.Table1.6Estimate of patents of the major companies active in Fischer-Tropsch(April 1998).BP1340Exxon71155Rentech180Sasol233Shell452713Statoil531Syntroleum01010C HAPTER1Table1.7Comparison of the major companies active in Fischer-Tropsch(October1998).Energy Int.PO with O2slurry-CoExxon CPO(O2)slurry200Co Rentech PO with O2,SR,ATR slurry235FeSasol PO with O2,SR,slurry2,500Fe,Cocoal gasificationfluidized110,000Shell2PO with O2fixed12,500Co Syntroleum ATR with airfixed2Cowas dismantled and transported to Arunachal,India were it is expected to produce 350bbl/day of waxes in1999in cooperation with the Indian company Donyi Polo Petrochemicals Ltd.SasolSasol has operated commercial Fischer-Tropsch plants since1955.A detailed review of Sasol’s commercial plants from1950to1979is given by Dry[32].A commercial plant in Sasolburg(South Africa)(Sasol1)use multitubular(2050tubes,50mm ID)fixed bed and entrained bed Kellogg reactors.Synthesis gas is predominantly pro-duced with Lurgi coal gasifiers.Sasol2and Sasol3plants in Secunda went on stream in the beginning of the eighties.These plants use circulatingfluidized bed reactors (Synthol,Figure1.4c)for the production of fuels and low molecular weight olefins. Currently,Sasol has two new processes for the Fischer-Tropsch synthesis.A process at high temperatures(HTFT:330-350˚C)for the production of gasoline and light olefins and a process for wax production at lower temperatures(LTFT:220-250˚C). The HTFT is performed in Synthol circulatingfluidized bed(CFB)reactors,but a more efficient Sasol Advanced Synthol(SAS)reactor with gas-solidfluidization was devel-oped recently[16].The Synthol reactors will be replaced by the new SAS reactors. Conventionally,ARGE tubularfixed bed reactors were used for the LTFT process. In1990,a slurry bubble column reactor(Sasol Slurry Phase Distillate;SSPD)with a diameter of1m was commissioned[15].A commercial-scale slurry reactor is in operation since1993and has a diameter of5m and a height of22m with a capacity of about2,500bbl/day.Table1.8shows an overview of different Sasol reactors[15,19]. Further scale up of the SSPD reactor is planned to20,000bbl/day per reactor.Table1.8Sasol Fischer-Tropsch commercial reactors(bbl/day),adapted from Jager[19].Total installed capacity1100001100032002500 Capacity per reactor650011000500-7002500Potential per reactor750020000300020000technology in Nigeria.The estimated cost price of this complex is$500-600million (Remote Gas Strategies,May1998).Most patents of Sasol(see Table1.6)concern the development of a slurry reactor with continuous in-situ wax-solid separation[33]and grade-up of olefins by hydroformulation[34].ShellIn1993,Shell started up a$850million FT synthesis plant in Bintulu,Malaysia.The Shell Middle Distillate Synthesis(SMDS)process[5,35]produces heavy paraffins on a cobalt catalyst in multitubular trickle bed reactors.Part of these products are sold as wax specialties;another part is hydro-cracked over a noble metal catalyst into clean transportation fuels(see Table1.5).The plant converts100million cubic feet/day of natural gas from off-shorefields by non-catalytic partial oxidation into12,500bbl/day hydrocarbons.The air separation plant of the SMDS plant in Bintulu exploded in December1997.Shell Oil wants to reopen the SMDS plant in1999(Remote Gas Strategies,April1998).Most Shell patents focus on either catalyst development or on the way the SMDS process is preferably carried out,for example,see patents[36,37]. Some patents for improving a slurry process have beenfiled as well[38–40].StatoilPatents of Statoil involve slurry reactor design and continuous catalyst-wax separations with the use offiltration[41].Recent patents with respect to Fischer-Tropsch catalysis concern the development of cobalt catalysts promoted with Rh,Pt,Ir,or Re on alumina (for example,[42]).Statoil formed an alliance with Sasol for the development of floating Fischer-Tropsch plants on ships orfloating production systems.Thesefloating off-shore plants can be used to utilize natural gas associated with oil production[43].SyntroleumSyntroleum is a small researchfirm in Tulsa,Oklahoma,USA,which has signed li-censing agreements with Texaco,ARCO,Kerr-McGee,and Enron.A laboratory pilot plant(2bbl/day)is used to demonstrate their FT process.They claim that their pro-cess eliminates a costly air separation unit,since their Autothermal Reformer(ATR) produces nitrogen-diluted synthesis gas from natural gas[44].Nitrogen can be used to remove some of the generated heat during the FT reaction.The Syntroleum process is the basis of an agreement between Texaco,Brown&Root and Syntroleum to developa2,500bbl/day GTL plant,starting end1999(Remote Gas Strategies,January1998). Recently,Syntroleum and Enron announcedfinal agreement to build a8,000bbl/day GTL plant in Wyoming,USA.The plant is expected to operate in2001[45].1.3Research on the Fischer-Tropsch SynthesisAn optimal design with respect to product yield and selectivity of a large scale reactor requires a deep understanding of hydrodynamics,reaction kinetics,catalytic system and FT chemistry(see Figure1.5).Research on the various aspects of the FT process will be discussed briefly.A detailed review on kinetics and selectivity of the Fischer-Tropsch process is given in Chapter2.Figure1.5Modeling of a large scale Fischer-Tropsch reactor.Reaction KineticsThe complexity of the FT reaction mechanism and the large number of species in-volved is the major problem for development of reliable kinetic expressions.Most catalyst studies aim at catalyst improvement and postulate empirical power law kinet-ics for both the carbon monoxide conversions and the carbon dioxide formation rate [46,47].Langmuir-Hinshelwood-Hougen-Watson(LHHW)type of rate equations have been applied in literature(see Chapter2.8).The water gas shift reaction can play a dominant role on iron catalysts.Only a few studies report on WGS kinetics oniron catalysts under FT conditions.A thorough comparison of the available literature models is presented in Chapter2.Product SelectivityThe products from the Fischer-Tropsch synthesis form a complex multicomponent mixture with substantial variation in carbon number and product type.Main products are linear paraffins and-olefins.According to Anderson[48],the product distribu-tion of hydrocarbons can be described by the Anderson-Schulz-Flory(ASF)equation: m n1n1with m n the mole fraction of a hydrocarbon with chain length n and the growth probability factor independent of n.determines the total carbon number distribution of the FT products.The range of depends on reaction condi-tions and catalyst type.Dry[49]reported typical ranges of on Ru,Co,and Fe of: 0.85-0.95,0.70-0.80,and0.50-0.70,respectively.More recent references report Co catalysts with chain growth factors between0.85-0.95[5].Significant deviations from the ASF distribution are reported in literature:i)Relatively high yield of methane.ii) Relatively low yield of ethene.iii)Change in chain growth parameter and expo-nential decrease of the olefin to paraffin ratio with increasing carbon number.These deviations are predominantly caused by secondary reactions of-olefins,which may readsorb on growth sites of the catalyst surface and continue to grow via propagation with monomer or terminate as hydrocarbon product.Details on the characteristics of the product selectivity and on modeling of the selectivity are discussed in Chapter2. Reactor Engineering ModelMathematical modeling of FT slurry bubble columns was reviewed by Saxena et al.[17]and more recently by Saxena[50].He showed that none of the available mod-els is accurate enough for a reliable reactor design.The bottleneck appears to be the lack of reliable kinetic equations for all products and reactants based on realistic re-action mechanisms.Until now,none of the available literature models obtain enough details to describe the complete product distribution of the Fischer-Tropsch synthesis at industrial conditions(high temperature and pressure)as a function of overall con-sumption of synthesis gas components and operating conditions.Either the product distribution model(ASF behavior)or the kinetic scheme(no WGS and rates equa-tions withfirst order in hydrogen)is oversimplified,or the hydrodynamic situation is unrealistic under industrial(churn-turbulent or heterogeneousflow regime)operating conditions.The features of the models available will be compared in Chapter7.1.4Aims and Outline of this ThesisThe problem to be dealt with in this thesis is the lack of accurate models for prod-uct distributions and reaction kinetics,necessary for reliable design and scale up of industrial Fischer-Tropsch processes.Therefore,the major aim of this thesis is the development of a product distribution model and a kinetic model both in gas-slurry as well as in gas-solid reactors over a commercial precipitated iron catalyst based on own experimental work.The product distribution model should be able to explain the deviations from the ASF distribution observed experimentally.It should include a mechanistic model of olefin readsorption and kinetics of chain growth and termination on the same catalytic sites.Accurate intrinsic rate expressions for the CO conversion to Fischer-Tropsch products and for the water gas shift(WGS)reaction over a precipitated iron catalyst on the basis of reliable mechanisms are another aim.A detailed multicomponent mathematical model for a large scale slurry bubble column reactor with use of our detailed models is the final aim of this thesis.Chapter2presents a literature review on the kinetics and selectivity of the Fischer-Tropsch synthesis.The focus is on the reaction mechanisms and kinetic models of the water gas shift and Fischer-Tropsch reactions.Literature product selectivity models are reviewed as well.Here the areas which require further research will be defined.Chapter3describes the experimental setup of the kinetic experiments both in a gas-solid and gas-slurry laboratory kinetic reactor and the catalyst applied.The ana-lytical section and the experimental procedures are described as well.The development of a new-Olefin Readsorption Product Distribution Model (ORPDM)based on own experiments for the gas-solid FT synthesis,over a precip-itated iron catalyst is presented in Chapter4.The effect of variation of process condi-tions on the selectivity is described as well.Chapter5presents the kinetic experiments and kinetic modeling of the CO hydro-genation and the water gas shift reaction of gas-solid Fischer-Tropsch synthesis over the precipitated iron catalyst.The influence of the slurry liquid on the product selectivity and the reaction ki-netics is presented in Chapter6.The product selectivity model developed for the gas-solid system will be applied for the description of the product selectivity at in-dustrially relevant conditions over a precipitated iron catalyst suspended in the slurry phase.Furthermore,Chapter6describes kinetic modeling of the gas-slurry Fischer-Tropsch synthesis based on a methodology derived in Chapter5.The models obtained in Chapters4-6and literature data on hydrodynamics and mass transfer in the heterogeneousflow regime are incorporated in a multicomponent reaction engineering model for a large scale Fischer-Tropsch slurry bubble column reactor in Chapter7.The main novel aspect of this model is that,for thefirst time, multicomponent vapor-liquid equilibria with detailed kinetic expressions for all reac-tants and products(based on original experimental work)are combined to predict the compositions of the gaseous and liquid streams and the performance of a slurry bubble column reactor.References[1]Kroschwitz,I.;Howe-Grant,M.,Kirk-Othmer encyclopedia of chemical tech-nology,Wiley&Sons,New York,fourth edn.1996.[2]Fischer,F.;Tropsch,H.,Uber die Herstellung synthetischer¨o lgemische(Syn-thol)durch Aufbau aus Kohlenoxyd und Wasserstoff,Brennst.Chem.1923,4, 276–285.[3]Fischer,F.;Tropsch,H.,German Patent4843371925.[4]Jager,B.;Espinoza,R.,Advances in low-temperature Fischer-Tropsch synthesis,Catal.Today1995,23,17–28.[5]Sie,S.T.,Process development and scale up:IV Case history of the developmentof a Fischer-Tropsch synthesis process,Rev.Chem.Eng.1998,14,109–157. [6]Choi,G.N.;Kramer,S.J.;Tam,S.T.;Fox,J.M.,Design/economics of a naturalgas based Fischer-Tropsch plant,in Spring National Meeting,American Institute of Chemical Engineers,Houston,1996.[7]Cybulski, A.;Edvinsson,R.;Irandoust,S.;Andersson, B.,Liquid-phasemethanol synthesis:modelling of a monolithic reactor,Chem.Eng.Sci.1993, 48,3463–3478.[8]Perry,R.H.;Green,D.,Perry’s chemical engineers‘handbook,McGraw-Hill,New York,6th edn.1984.[9]Basini,L.;Piovesan,L.,Reduction on synthesis gas costs by decrease ofsteam/carbon and oxygen/carbon ratios in the feedstock,Ind.Eng.Chem.Res.1998,37,258–266.[10]Rentech,Gas to liquids home page,/tech2.htm(ac-cessed December1998).[11]Rao,V.U.S.;Stiegel,G.J.;Cinquegrane,G.J.;Srivastave,R.D.,Iron-based cat-alysts for slurry-phase Fischer-Tropsch process:Technology review,Fuel Pro-cess.Technol.1992,30,83–107.[12]Xu,L.;Bao,S.;R.J.,O’Brien;Raje,A.;Davis,B.H.,Don’t rule out iron catalystsfor Fischer-Tropsch synthesis,CHEMTECH1998,8,47–53.[13]Raje,A.P.;Davis,B.H.,Fischer-Tropsch synthesis:process considerations basedon performance of iron-based catalysts,Fuel1997,76,273–280.[14]Udovich,C.A.,Ceramic membrane reactors for the conversion of natural gas tosyngas,Stud.Surf.Sci.Catal.1998,119,417–422.[15]Jager,B.,Developments in Fischer-Tropsch technology,Stud.Surf.Sci.Catal.1997,107,219–224.[16]Jager,B.;Dry,M.E.;Shingles,T.;Steynberg,A.P.,Experience with a new typeof reactor for Fischer-Tropsch synthesis,Catal.Lett.1990,7,293–302. [17]Saxena,S.C.;Rosen,M.;Smith,D.N.;Ruether,J.A.,Mathematical modeling ofFischer-Tropsch slurry bubble column reactors,mun.1986,40, 97–151.[18]De Swart,J.W.A.,Scale-up of a Fischer-Tropsch reactor,Ph.D.thesis,Univer-sity of Amsterdam,Amsterdam,The Netherlands1996.[19]Jager,B.,Developments in Fischer-Tropsch technology,Stud.Surf.Sci.Catal.1998,119,25–34.[20]Choi,G.N.;Kramer,S.J.;Tam,S.T.;Fox,J.M.;Carr,N.L.;Wilson,G.R.,De-sign/economics of a once-through natural gas based Fischer-Tropsch plant with power co-production,in Coal liquefaction and solid fuels,Pittsburgh,1997. [21]Fox,III,J.M.,The different catalytic routes for methane valorization:an assess-ment of processes for liquid fuels,Catal.Rev.-Sci.Eng.1993,35,169–212. [22]Gregor,J.H.,Fischer-Tropsch products as liquid fuels or chemicals.An econom-ical evaluation,Catal.Lett.1990,7,317–332.[23]Singleton,A.H.,Advances make gas-to-liquids process competitive for remotelocations,Oil Gas J.1997,68–72.[24]Eisenberg,B.;Fiato,R.A.;Mauldin,C.H.;Say,G.R.;Soled,S.L.,Exxon’s ad-vanced gas-to-liquids technology,Stud.Surf.Sci.Catal.1998,119,943–948. [25]Davis,B.H.,Fischer-Tropsch conversion of gas to liquid,Appl.Catal.A1997,155,N4–N7.[26]Behrmann,W.C.;Mauldin,C.H.;Pedrick,L.E.,Patent WO94147351994,Exxon.[27]Koros,R.B.,US Patent53843361995,Exxon.[28]Benham,C.B.;Bohn,M.S.;Yakobson,D.L.,US Patent55041181996,Rentech.[29]Benham,C.B.;Bohn,M.S.;Yakobson,D.L.,US Patent56206701997,Rentech.[30]Benham,C.B.;Bohn,M.S.;Yakobson,D.L.,US patent56211551997,Rentech.[31]Benham,C.B.;Bohn,M.S.;Yakobson,D.L.,US Patent55344371996,Rentech.[32]Dry,M.E.,The Fischer-Tropsch synthesis,in J.R.Anderson;M.Boudart,eds.,Catalysis-Science and technology,vol.1,Springer-Verlag,New York,1981pp.160–255.[33]Inga,J.R.;Jager,B.;Kelfkens,R.C.;Malherbe,F.E.J.;Smith,M.A.;Steynberg,A.P.,Eur.Patent609079,US patent55998491994,Sasol.[34]Betts,M.J.Dry,M.E.;Geertsema,A.;Rall,G.J.H.,Patent WO97015211997,Sasol.[35]Sie,S.T.;Senden,M.M.G.;Van Wechum,H.M.H.,Conversion of natural gas totransportation fuels via the Shell Middle Destillate Synthesis process(SMDS), Catal.Today1991,8,371–394.[36]Bode,D.;Sie,S.T.,Eur.Patent1883041986,Shell.[37]Post,M.F.M.;Sie,S.T.,Eur.Patent1672151984,Shell.[38]Engel,D.C.;Van der Honing,G.,Patent WO97316931997,Shell.[39]Wijn,E.F.;Danckaarts,A.M.,Eur.Patent6943251996,Shell.[40]McEwan,M.W.;Teekens,M.B.,US Patent6943251978,Shell.[41]Lorentzen,G.B.;Myrstad,T.;Westvik,A.,US Patent55208901995,Statoil.[42]Eri,S.;Goodwin,J.G.;Marcelin,G.;Riis,T.,US Patent48015731989,Statoil.[43]Statoil,Press release April14,1997.[44]Agee,K.L.;Willingham,F.Y.,WO Patent97338471997,Syntroleum.[45]Syntroleum,Press release February24,1998.[46]Bub,G.;Baerns,M.,Prediction of the performance of catalyticfixed bed reactorsfor Fischer-Tropsch synthesis,Chem.Eng.Sci.1980,35,348–355.[47]Newsome,D.S.,The water-gas shift reaction,Catal.Rev.-Sci.Eng.1980,21,275–318.[48]Anderson,R.B.,Catalysts for the Fischer-Tropsch synthesis,vol.4,Van Nos-trand Reinhold,New York1956.[49]Dry,M.E.,Catalytic aspects of industrial Fischer-Tropsch synthesis,J.Mol.Catal.1982,17,133–144.[50]Saxena,S.C.,Bubble column reactors and Fischer-Tropsch synthesis,Catal.Rev.-Sci.Eng.1995,37,227–309.。
甲烷重整制氢工艺流程

甲烷重整制氢工艺流程Methane reforming is a process that converts methane, the primary component of natural gas, into hydrogen. This process is crucial for the production of hydrogen, which is used in various industries and applications worldwide. 甲烷重整是将天然气的主要成分甲烷转化为氢气的过程。
这个过程对氢气的生产至关重要,氢气在世界各个行业和应用中被广泛使用。
One of the most common methods for methane reforming is steam methane reforming (SMR), which involves reacting methane with steam in the presence of a catalyst to produce hydrogen and carbon monoxide. Another method is autothermal reforming (ATR), which combines steam reforming with partial oxidation to produce hydrogen and carbon monoxide. These processes play a significant role in the production of hydrogen for various industrial applications. 甲烷重整的最常见方法之一是蒸汽甲烷重整(SMR),它涉及在催化剂的存在下将甲烷与水蒸气反应,产生氢气和一氧化碳。
另一种方法是自热重整(ATR),它将蒸汽重整与部分氧化相结合,生成氢气和一氧化碳。
甲醇自热重整 英文

甲醇自热重整英文Methanol self-thermal reforming is a process that involves the conversion of methanol into hydrogen and carbon dioxide. This process is carried out in the presence of a catalyst and requires heat to drive the reaction forward. The hydrogen produced can then be used as a fuel in various applications, such as fuel cells or internal combustion engines.One of the advantages of methanol self-thermal reforming is that it can be carried out at relatively low temperatures, which makes it more energy-efficient compared to other hydrogen production methods. Additionally, methanol is a readily available and relatively inexpensive feedstock, which makes this process economically viable.However, there are also some challenges associated with methanol self-thermal reforming. One of the main challenges is the development of efficient catalysts that can operate at low temperatures and have high selectivity for hydrogen production. Another challenge is the management of the heat generated during the reaction, as this can affect the efficiency and stability of the process.Despite these challenges, methanol self-thermal reforming holds great promise as a sustainable and efficient method for hydrogen production. Ongoing research and development efforts are focused onaddressing these challenges and improving the performance and scalability of this technology. With continued advancements, methanol self-thermal reforming could play a significant role in the transition to a hydrogen-based economy.。
甲烷裂解催化剂al2o3的钯

甲烷裂解催化剂al2o3的钯英文回答:Methane decomposition catalysts play a crucial role in various industrial processes, such as the production of hydrogen and carbon nanotubes. One widely used catalyst for methane decomposition is palladium supported on alumina (Pd/Al2O3). In this response, I will discuss the properties and performance of Pd/Al2O3 catalysts and provide examples to illustrate their effectiveness.Pd/Al2O3 catalysts exhibit excellent catalytic activity and selectivity for methane decomposition due to the unique properties of both palladium and alumina. Palladium is known for its ability to dissociate methane into hydrogen and carbon, while alumina provides a high surface area and thermal stability. The combination of these two materials creates an optimal environment for the catalytic reaction.One advantage of Pd/Al2O3 catalysts is their highactivity, which allows for efficient methane decomposition at relatively low temperatures. For example, at temperatures around 500°C, Pd/Al2O3 catalysts can achieve a methane conversion rate of over 90%. This high activity is attributed to the strong metal-support interaction between palladium and alumina, which enhances the dispersion and accessibility of palladium active sites.Another important characteristic of Pd/Al2O3 catalysts is their selectivity towards hydrogen production. Methane decomposition can result in the formation of undesirable by-products, such as carbonaceous deposits or carbon monoxide. However, Pd/Al2O3 catalysts exhibit a high selectivity towards hydrogen, minimizing the formation of these by-products. This selectivity is crucial for applications where pure hydrogen is required, such as fuel cells.Furthermore, Pd/Al2O3 catalysts demonstrate good stability and resistance to deactivation. The alumina support provides thermal stability, allowing the catalyst to maintain its activity even at high temperatures.Additionally, the strong metal-support interaction prevents the aggregation or sintering of palladium particles, ensuring the long-term stability of the catalyst.To illustrate the effectiveness of Pd/Al2O3 catalysts, let's consider an example. Imagine a hydrogen production plant that utilizes methane decomposition to generate hydrogen gas. The plant requires a catalyst that can efficiently convert methane into hydrogen at a low temperature to minimize energy consumption. By using aPd/Al2O3 catalyst, the plant can achieve high conversion rates and selectivity towards hydrogen, resulting in acost-effective and environmentally friendly process.中文回答:甲烷裂解催化剂Al2O3的钯在各种工业过程中起着至关重要的作用,例如氢气和碳纳米管的生产。
烟气抗硫脱汞材料的研究进展

第52卷第1期2021年1月中南大学学报(自然科学版)Journal of Central South University (Science and Technology)V ol.52No.1Jan.2021烟气抗硫脱汞材料的研究进展沈畅1,2,王卉2,沈昊天2,吴建飞2(1.南京理工大学钱学森学院,江苏南京,210094;2.南京理工大学能源与动力工程学院,江苏南京,210094)摘要:综述了近年来烟气抗硫脱汞材料的研究进展,提出抗硫脱汞材料设计的4种方法,分别为活性位保护法、活性位增加法、氧活性位规避法和贵金属催化法。
活性位的保护可以利用材料的亲硫性或抗硫性来实现,活性位的增加可以通过表面氧缺陷构造或者气氛调控来实现,氧活性位的规避可以通过抗硫活性位的设计来实现,而贵金属催化剂的抗硫脱汞机理则复杂多变。
这4种方法的提出将为抗硫脱汞材料的进一步研发提供参考。
关键词:脱汞;烟道气;抗硫;活性位;催化剂;吸附剂中图分类号:X511文献标志码:A开放科学(资源服务)标识码(OSID)文章编号:1672-7207(2021)01-0133-11Research progress on flue gas mercury removal materials withSO 2resistanceSHEN Chang 1,2,WANG Hui 2,SHEN Haotian 2,WU Jianfei 2(1.Tsien Hsue −shen College,Nanjing University of Science and Technology,Nanjing 210094,China;2.School of Energy and Power Engineering,Nanjing University of Science and Technology,Nanjing 210094,China)Abstract:The recent research progress on mercury removal materials with SO 2resistance was reviewed.Four methods for the design of mercury removal materials with SO 2resistance were proposed,which were active site protection method,active site increase method,oxygen −containing active site evasion method and noble metal catalysts method.The protection of active sites can be achieved by doping the materials with sulfur affinity or sulfur resistance.The increase of active sites can be achieved by the enrichment of surface oxygen defects or theDOI:10.11817/j.issn.1672-7207.2021.01.013收稿日期:2020−06−01;修回日期:2020−09−05基金项目(Foundation item):国家自然科学基金资助项目(51706104);江苏省自然科学基金资助项目(BK20170849);江苏省六大人才高峰项目(XNY-026);南京理工大学大型仪器设备开放基金资助项目(60)(Project(51706104)supported by the National Natural Science Foundation of China;Project(BK20170849)supported by the Natural Science Foundation of Jiangsu Province;Project(XNY-026)supported by the Peak of the Six Talents Program of Jiangsu Province;Project(60)supported by the Open Fund for Large-scale Equipments of NUST)通信作者:王卉,博士,副教授,从事燃煤汞污染物控制研究;E −mail:*******************.cn引用格式:沈畅,王卉,沈昊天,等.烟气抗硫脱汞材料的研究进展[J].中南大学学报(自然科学版),2021,52(1):133−143.Citation:SHEN Chang,WANG Hui,SHEN Haotian,et al.Research progress on flue gas mercury removal materials with SO 2resistance[J].Journal of Central South University(Science and Technology),2021,52(1):133−143.第52卷中南大学学报(自然科学版)adjustment of reaction atmosphere.The evasion of oxygen−containing active sites can be achieved through thedesign of SO2−resistant active sites.The mechanisms of mercury removal with SO2resistance of noble metal catalysts are complicated which can vary with certain materials.The proposed four methods will provide thetheoretical reference for further development of mercury removal materials with SO2resistance.Key words:mercury removal;flue gas;SO2resistance;active site;catalyst;adsorbent汞是一种具有高挥发性、强神经毒性、持续生物累积性等特性的元素[1−3]。
常压下甲醇_碳酸二甲酯汽液平衡测定及其萃取剂选择

第39卷第8期2011年8月化学工程CHEMICAL ENGINEERING (CHINA )Vol.39No.8Aug.2011收稿日期:2011-03-11基金项目:国家自然科学基金资助项目(20476005);国家科技支撑计划课题子课题(2006BA109B07-01)作者简介:李群生(1963—),男,博士,教授,从事精馏、吸收、超临界萃取、连续结晶理论与技术的研究,E-mail :liqsh@buct.edu.cn ;朱炜,通讯联系人,E-mail :zw198204@163.com 。
常压下甲醇-碳酸二甲酯汽液平衡测定及其萃取剂选择李群生1,朱炜1,付永泉1,高东江2,王海川1,王浩1,李仑1(1.北京化工大学化学工程学院,北京100029;2.兰州新西部维尼龙有限公司,甘肃兰州730094)摘要:甲醇(MeOH )与尿素催化合成碳酸二甲酯的工艺路线是目前最有发展前途的一种合成方法,但该过程中由于使用了过量的甲醇,在合成中形成了碳酸二甲酯和甲醇的共沸物,分离困难。
已报道的分离方法中萃取精馏,在经济效益、操作和安全方面都优于其他方法。
草酸二甲酯,碳酸乙烯酯,碳酸丙烯酯均可作为萃取精馏分离DMC 的萃取剂,但MeOH-DMC-萃取剂萃取精馏的数学模型尚未见报道,所以有必要研究MeOH-DMC 及其与萃取剂体系的汽液平衡数据。
本文在常压下,用改进的Othmer 釜测定了MeOH-DMC 二元汽液平衡数据,并用Margules ,Van Laar ,Wilson ,NRTL ,UNIQUAC 方程对实验数据进行了关联,并利用UNIFAC 方程模拟推算了MeOH-DMC-萃取剂(草酸二甲酯、碳酸乙烯酯、碳酸丙烯酯)三元体系在常压下的汽液平衡,为建立萃取精馏法分离DMC 与甲醇共沸体系的数学模型提供了必要的汽液平衡(VLE )数据。
关键词:碳酸二甲酯;甲醇;萃取剂;汽液平衡中图分类号:TQ 013.1文献标识码:A文章编号:1005-9954(2011)08-0044-04Vapor-liquid equilibrium of methanol-dimethyl carbonate atnormal pressure and selection of extractantLI Qun-sheng 1,ZHU Wei 1,FU Yong-quan 1,GAO Dong-jiang 2,WANG Hai-chuan 1,WANG Hao 1,LI Lun 1(1.College of Chemical Engineering ,Beijing University of Chemical Technology ,Beijing 100029,China ;2.Lanzhou New West Vinylon Company Limited ,Lanzhou 730094,Gansu Province ,China )Abstract :The route of catalytic synthesizing dimethyl carbonate by methanol (MeOH )and urea is the most promising synthetic method.But because of the excessive use of methanol ,it is difficult to separate dimethyl carbonate and methanol mixture due to the existence of azeotrope in this process.Extractive distillation is more economical ,simple ,safe and effective than the other separation methods reported ,and dimethyl oxalate (DMO ),ethylene carbonate (EC )and propylene carbonate (PC )can be used as the extractant.However ,the mathematical model of MeOH-DMC-extractant was not reported.So ,it is necessary to study the vapor-liquid equilibrium (VLE )data of MeOH-DMC and its extractant.The vapor-liquid equilibrium data for the dimethyl carbonate and methanol were measured at normal pressure in a modified Othmer still.The measured binary data were correlated by using Margules ,Van Laar ,Wilson ,NRTL and UNIQUAC models.The normal atmosphere vapor-liquid equilibrium of ternary system MeOH-DMC-extractant (dimethyl oxalate ,ethylene carbonate ,propylene carbonate )was simulated by using the UNIFAC equation.The VLE data are necessary to separate methanol from DMC by extraction distillation.Key words :dimethyl carbonate ;methanol ;extractant ;vapor-liquid equilibrium 碳酸二甲酯[1-6](DMC )是绿色化工产品,由于其分子中含有羰基、甲氧基和甲基等多种官能团,因此,在有机合成中可作为甲基化试剂代替原来有致癌作用的硫酸二甲酯广泛用于合成农药、医药和燃料等化工生产中。
车载甲醇重整制氢燃料电池系统建模与供氢管理
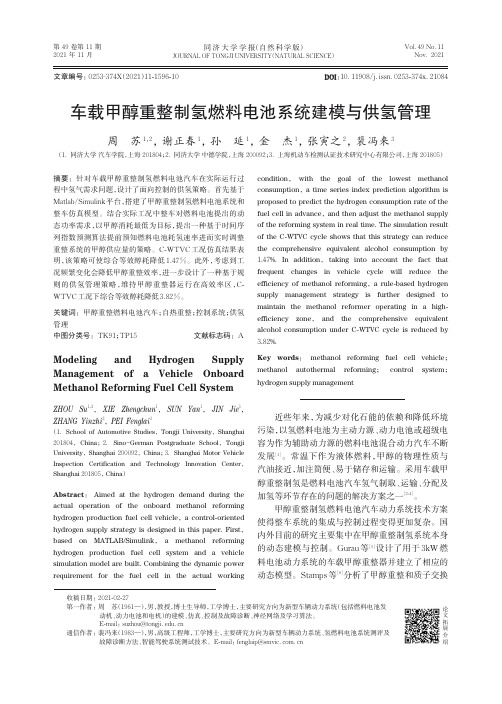
第49卷第11期2021年11月同济大学学报(自然科学版)JOURNAL OF TONGJI UNIVERSITY(NATURAL SCIENCE)Vol.49No.11Nov.2021论文拓展介绍车载甲醇重整制氢燃料电池系统建模与供氢管理周苏1,2,谢正春1,孙延1,金杰1,张寅之2,裴冯来3(1.同济大学汽车学院,上海201804;2.同济大学中德学院,上海200092;3.上海机动车检测认证技术研究中心有限公司,上海201805)摘要:针对车载甲醇重整制氢燃料电池汽车在实际运行过程中氢气需求问题,设计了面向控制的供氢策略。
首先基于Matlab/Simulink平台,搭建了甲醇重整制氢燃料电池系统和整车仿真模型。
结合实际工况中整车对燃料电池提出的动态功率需求,以甲醇消耗最低为目标,提出一种基于时间序列指数预测算法提前预知燃料电池耗氢速率进而实时调整重整系统的甲醇供应量的策略。
C-WTVC工况仿真结果表明,该策略可使综合等效醇耗降低1.47%。
此外,考虑到工况频繁变化会降低甲醇重整效率,进一步设计了一种基于规则的供氢管理策略,维持甲醇重整器运行在高效率区,C-WTVC工况下综合等效醇耗降低3.82%。
关键词:甲醇重整燃料电池汽车;自热重整;控制系统;供氢管理中图分类号:TK91;TP15文献标志码:A Modeling and Hydrogen Supply Management of a Vehicle Onboard Methanol Reforming Fuel Cell System ZHOU Su1,2,XIE Zhengchun1,SUN Yan1,JIN Jie1,ZHANG Yinzhi2,PEI Fenglai3(1.School of Automotive Studies,Tongji University,Shanghai 201804,China;2.Sino-German Postgraduate School,Tongji University,Shanghai200092,China;3.Shanghai Motor Vehicle Inspection Certification and Technology Innovation Center,Shanghai201805,China)Abstract:Aimed at the hydrogen demand during the actual operation of the onboard methanol reforming hydrogen production fuel cell vehicle,a control-oriented hydrogen supply strategy is designed in this paper.First,based on MATLAB/Simulink,a methanol reforming hydrogen production fuel cell system and a vehicle simulation model are bining the dynamic power requirement for the fuel cell in the actual working condition,with the goal of the lowest methanolconsumption,a time series index prediction algorithm isproposed to predict the hydrogen consumption rate of thefuel cell in advance,and then adjust the methanol supplyof the reforming system in real time.The simulation resultof the C-WTVC cycle shows that this strategy can reducethe comprehensive equivalent alcohol consumption by 1.47%.In addition,taking into account the fact that frequent changes in vehicle cycle will reduce theefficiency of methanol reforming,a rule-based hydrogensupply management strategy is further designed tomaintain the methanol reformer operating in a high-efficiency zone,and the comprehensive equivalentalcohol consumption under C-WTVC cycle is reduced by 3.82%.Key words:methanol reforming fuel cell vehicle;methanol autothermal reforming;control system;hydrogen supply management近些年来,为减少对化石能的依赖和降低环境污染,以氢燃料电池为主动力源、动力电池或超级电容为作为辅助动力源的燃料电池混合动力汽车不断发展[1]。
甲醇水相重整制氢原位还原苯乙酮制备苯乙醇
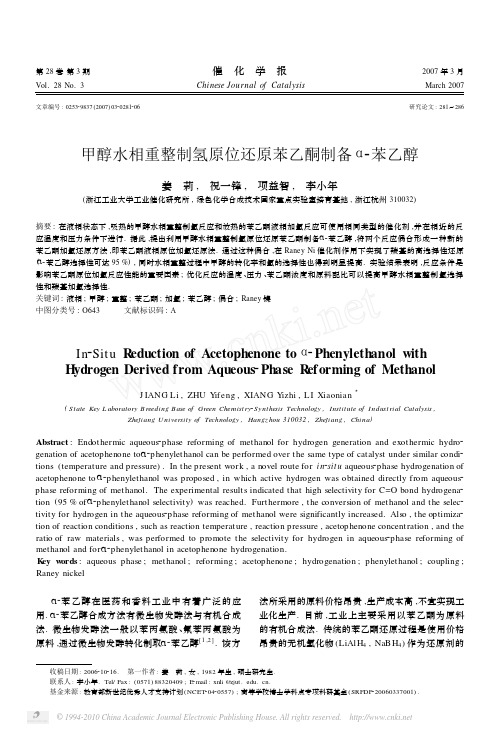
文章编号:025329837(2007)0320281206研究论文:281~286收稿日期:2006210216. 第一作者:姜 莉,女,1982年生,硕士研究生.联系人:李小年.Tel/Fax :(0571)88320409;E 2mail :xnli @.基金来源:教育部新世纪优秀人才支持计划(NCET 20420557);高等学校博士学科点专项科研基金(SRFDP 220060337001).甲醇水相重整制氢原位还原苯乙酮制备α2苯乙醇姜 莉, 祝一锋, 项益智, 李小年(浙江工业大学工业催化研究所,绿色化学合成技术国家重点实验室培育基地,浙江杭州310032)摘要:在液相状态下,吸热的甲醇水相重整制氢反应和放热的苯乙酮液相加氢反应可使用相同类型的催化剂,并在相近的反应温度和压力条件下进行.据此,提出利用甲醇水相重整制氢原位还原苯乙酮制备α2苯乙醇,将两个反应偶合形成一种新的苯乙酮加氢还原方法,即苯乙酮液相原位加氢还原法.通过这种偶合,在Raney Ni 催化剂作用下实现了羰基的高选择性还原(α2苯乙醇选择性可达95%),同时水相重整过程中甲醇的转化率和氢的选择性也得到明显提高.实验结果表明,反应条件是影响苯乙酮原位加氢反应性能的重要因素;优化反应的温度、压力、苯乙酮浓度和原料配比可以提高甲醇水相重整制氢选择性和羰基加氢选择性.关键词:液相;甲醇;重整;苯乙酮;加氢;苯乙醇;偶合;Raney 镍中图分类号:O643 文献标识码:AIn 2Situ R eduction of Acetophenone to α2Phenylethanol withH ydrogen Derived from Aqueous 2Phase R eforming of MethanolJ IANG Li ,ZHU Y ifeng ,XIANG Y izhi ,L I Xiaonian3(S tate Key L aboratory B reeding B ase of Green Chemist ry 2Synthesis Technology ,Institute of Indust rial Catalysis ,Zhejiang U niversity of Technology ,Hangz hou 310032,Zhejiang ,China )Abstract :Endothermic aqueous 2phase reforming of methanol for hydrogen generation and exothermic hydro 2genation of acetophenone to α2phenylethanol can be performed over the same type of catalyst under similar condi 2tions (temperature and pressure ).In the present work ,a novel route for i n 2sit u aqueous 2phase hydrogenation of acetophenone to α2phenylethanol was proposed ,in which active hydrogen was obtained directly from aqueous 2phase reforming of methanol.The experimental results indicated that high selectivity for C=O bond hydrogena 2tion (95%of α2phenylethanol selectivity )was reached.Furthermore ,the conversion of methanol and the selec 2tivity for hydrogen in the aqueous 2phase reforming of methanol were significantly increased.Also ,the optimiza 2tion of reaction conditions ,such as reaction temperature ,reaction pressure ,acetophenone concentration ,and the ratio of raw materials ,was performed to promote the selectivity for hydrogen in aqueous 2phase reforming of methanol and for α2phenylethanol in acetophenone hydrogenation.K ey w ords :aqueous phase ;methanol ;reforming ;acetophenone ;hydrogenation ;phenylethanol ;coupling ;Raney nickel α2苯乙醇在医药和香料工业中有着广泛的应用.α2苯乙醇合成方法有微生物发酵法与有机合成法.微生物发酵法一般以苯丙氨酸、氟苯丙氨酸为原料,通过微生物发酵转化制取α2苯乙醇[1,2].该方法所采用的原料价格昂贵,生产成本高,不宜实现工业化生产.目前,工业上主要采用以苯乙酮为原料的有机合成法.传统的苯乙酮还原过程是使用价格昂贵的无机氢化物(LiAlH 4,NaBH 4)作为还原剂的第28卷第3期催 化 学 报2007年3月Vol.28No.3Chi nese Journal of CatalysisMarch 2007计量还原法,该过程产生大量含有金属离子的废液,不仅增加了产物的分离难度,而且造成了环境污染[3].环境友好的催化还原技术是由芳香酮类化合物制备芳香醇的主要方法[4].均相催化还原技术具有较高的加氢活性和选择性[5~8],但催化剂回收困难等影响了其工业化应用.使用固体催化剂催化苯乙酮液相加氢合成α2苯乙醇可以有效地解决这些问题.该反应通常在Raney Ni[9~11],非晶态Ni[12,13],以及负载型(常用载体为Al2O3,TiO2, SiO2,H2ZSM5,HY等)Pt[14~16],Ru[17,18],Pd[19]和Cu[20]等催化剂作用下,在甲醇[18,21]、乙醇[12,16]、环己烷[22]和正己烷[17]等溶剂中,利用外界提供的氢将苯乙酮还原成α2苯乙醇.文献[10, 22~25]对Raney Ni催化剂上苯乙酮选择加氢反应进行了详尽的研究,在优化条件下,苯乙酮转化率可达到100%,α2苯乙醇选择性为82%.文献[16]研究了在酸性Y型沸石负载单金属Ni及双金属Ni2 Pt催化剂作用下苯乙酮选择加氢反应中的溶剂效应,发现极性质子溶剂(如甲醇、乙醇、异丙醇)对提高反应活性有利;而非极性非质子溶剂(如正己烷)则有利于提高羰基加氢选择性,但同时也大大抑制了反应活性. Dumesic研究小组[26~28]提出由生物质的衍生物醇类和水,在Raney Ni,Pt和Pd等催化剂的作用下采用水相重整反应技术可一步制得氢(选择性近100%).我们也进行了类似的工作,得到了相同的结果[29].以甲醇为例,水相重整制氢过程的化学反应方程式如下:CH3OH+H2O CO2+3H2-Q1(1) 本文基于在液相状态下进行的吸热的醇类水相重整制氢反应和放热的苯乙酮液相催化加氢反应可使用相同类型的催化剂,并能在比较相近的反应温度和压力下进行(两者之间具有非常好的偶合条件),提出将甲醇水相重整反应中产生的氢原位用于苯乙酮液相催化加氢反应,实现水相重整制氢和液相催化加氢两个反应的偶合:PhCOMe+H2PhCH(OH)Me+Q2(2) 3PhCOMe+H2O+CH3OH3PhCH(OH)Me+CO2(3)考察了Raney Ni催化剂作用下,由溶剂甲醇和水发生水相重整制氢反应产生的活化氢原位还原苯乙酮合成α2苯乙醇反应的性能;比较了原位还原法与氢气还原法及转移加氢法在苯乙酮加氢制备α2苯乙醇反应中活性和选择性的差异;考察了反应温度、压力、空速、浓度和溶液配比等对苯乙酮液相原位加氢制备α2苯乙醇反应的影响.1 实验部分1.1 催化剂样品 Raney Ni催化剂的制备方法及其化学组成和物化参数参见文献[30].1.2 苯乙酮原位加氢反应 苯乙酮液相原位催化加氢制备α2苯乙醇反应在固定床反应器(内径8mm)中进行.在反应器中加入215g催化剂,按照n(PhCOMe)∶n(MeOH)∶n(H2O)=1∶(215~20)∶(20~80)投料(物料配比范围的确定必须保证反应物之间能够完全互溶),反应温度为393~433K,充入氩气调节系统压力升至高于进料的鼓泡点0105MPa以保证反应在液相下进行,混合液体的时空速率为011~212h-1.反应器出口的产物经气液分离后分别由在线福立9790型气相色谱仪和Agilent6890GC25973MS型气相色谱2质谱联用仪进行分析. 间歇反应器催化反应评价实验在200ml高压釜中进行.在反应釜中加入215g催化剂,50ml苯乙酮的甲醇溶液(0143mol/L).在通氢气置换空气3次,再通氢气到一定的压力,升温到指定温度后开始搅拌(搅拌速率800r/min).加氢反应开始1h 后开始降温,滤去催化剂,产物由Agilent6890GC2 5973MS型气相色谱2质谱联用仪进行分析.2 结果与讨论2.1 原位加氢和其他加氢技术的比较 在固定床管式反应器中比较了不同苯乙酮加氢还原方法制备α2苯乙醇反应的性能,结果列于表1.可以看出,在各种加氢还原法优化的反应条件下,以甲醇为溶剂用氢气还原时苯乙酮的转化率为6010%,α2苯乙醇的选择性6414%,主要副产物包括乙苯和环己基乙烷;使用异丙醇氢转移法还原时,苯乙酮的转化率为5010%,α2苯乙醇的选择性7110%,主要副产物为乙苯;通过原位加氢法得到苯乙酮的转化率为6614%,α2苯乙醇的选择性为9511%,仅含有少量的乙苯和α2环己基乙醇. 在间歇反应器中进一步考察了氢气压力对苯乙酮催化加氢反应性能的影响(见表1).在333K的282催 化 学 报第28卷表1 不同苯乙酮加氢还原方法的比较Table1 Hydrogenation performance of acetophenone with various methodsReactor Method Solvent TKpMPaX(PhCOMe)%Product selectivity(w/%)PhCH(OH)Me PhEt C6H11CH(OH)Me C6H11COMe C6H11EtTubal HR MeOH333H20.660.064.421.4 1.20 13.0 HR MeOH403H20.6100.0036.50063.5 ISH MeOH2H2O403Ar0.666.495.1 4.00.900 MCH MeOH403Ar0.660.759.439.20.50.20.7 TH i2PrOH333Ar0.150.071.025.1 2.8 1.10TH i2PrOH403Ar0.698.9 2.345.8 2.0049.9 TH i2PrOH2H2O403Ar0.6100.0097.300 2.7 Batch HR MeOH333H20.188.975.623.300 1.1 HR MeOH333H20.695.684.114.900 1.0 HR MeOH333H22.094.083.316.2000.5 HR MeOH353H20.9100.084.415.6000 HR MeOH2H2O333H20.654.282.817.2000TH i2PrOH333Ar0.191.830.965.1 1.8 2.20 ISH MeOH2H2O403Ar0.613.884.515.5000 Reaction conditions:c(PhCOMe)=0143mol/L,n(MeOH)/n(H2O)=4/3,n(i2PrOH)/n(H2O)=4/3,L HSV=0171h-1,t=1h. HR hydrogen reduction,ISH i n2sit u hydrogenation,TH transfer hydrogenation,MCH methanol cracking hydrogenation.反应温度下,当氢气压力分别为011,016和210 MPa时,苯乙酮的转化率分别为8819%,9516%和9410%,α2苯乙醇的选择性分别为7516%、8411%和8313%.由此可以看出,提高氢气压力对苯乙酮的转化率和目标产物的选择性有影响但没有决定性的作用.此外,在333K的反应温度和016MPa的反应压力下比较了“甲醇2水”和“甲醇”两种不同溶剂中的反应性能,发现苯乙酮的转化率分别为5412%和9516%,而α2苯乙醇的选择性分别为8218%和8411%.由此可知,在溶剂中水的存在对α2苯乙醇的选择性没有明显影响,但显著地降低了苯乙酮的转化率.综合分析上面的实验结果可以推定,通过甲醇水相重整反应产生的原位氢与由氢气分子活化得到的氢及由氢转移试剂提供的氢之间的还原苯乙酮的活性存在差异.原位氢的高加氢选择性和低氢解选择性为提高α2苯乙醇选择性提供了一个新的途径.另外,由于原位加氢反应体系中含有较多的水,对催化剂的性能有一定的影响,进而影响苯乙酮加氢反应速率. 在管式反应器中,还考察了通过催化甲醇裂解制氢原位还原苯乙酮制备α2苯乙醇的反应性能.苯乙酮的转化率为6017%,α2苯乙醇的选择性为5914%,主要副产物为乙苯.这说明通过甲醇裂解制氢并原位还原苯乙酮,不同于“苯乙酮2甲醇2水”反应体系中的氢来自甲醇和水之间的水相重整反应,即甲醇水相重整制氢过程与甲醇裂解制氢过程产生的氢对苯乙酮加氢的性能存在明显的差异.2.2 反应条件的影响2.2.1 反应温度的影响 表2为反应温度对苯乙酮加氢反应的影响.可以看出,随着反应温度的升高,苯乙酮的转化率逐渐升高,α2苯乙醇的选择性先升高后降低,副产物乙苯的选择性则先降低后升高.反应温度为403K 时,α2苯乙醇的选择性达到9014%;反应温度继续升高时,α2苯乙醇的选择性明显下降.这是由于反应温度较高时,烯丙位的官能团相对不稳定,α2苯乙醇发生分子内脱水生成苯乙烯,进一步加氢而生成乙苯[18].相对较低的反应温度有利于提高α2苯乙醇的选择性;然而,甲醇水相重整制氢是一个吸热反应过程,反应温度过低对甲醇水相重整制氢反应是不利的.因此,在苯乙酮原位加氢偶合反应体系中存在一个最佳的反应温度.表2 反应温度对苯乙酮加氢反应的影响Table2 Effect of reaction temperature on hydrogenationof acetophenoneTKp(Ar)MPaX(PhCOMe)%Product selectivity(w/%)PhCH(OH)Me PhEt C6H11CH(OH)Me 3930.4436.683.816.20 4030.5240.490.49.10.5 4130.7347.677.422.10.5 4230.9352.763.834.8 1.4 Reaction conditions:n(H2O)/n(CH3OH)=4/3,c(Ph2COMe)=0192mol/L,L HSV=0171h-1.2.2.2 反应压力的影响 表3为反应压力对苯乙酮加氢反应的影响.可以看出,随着反应压力的升高,苯乙酮的转化率和382第3期姜 莉等:甲醇水相重整制氢原位还原苯乙酮制备α2苯乙醇表3 反应压力对苯乙酮加氢反应的影响Table3 Effect of reaction pressure on hydrogenation of acetophenonep(Ar) MPa X(PhCOMe)%Product selectivity(w/%)PhCH(OH)Me PhEt C6H11CH(OH)Me0.4029.477.821.50.70.5240.490.49.10.50.6046.492.47.30.30.7041.784.914.80.3Reaction conditions:n(H2O)/n(CH3OH)=4/3,c(Ph2COMe)=0192mol/L,L HSV=0171h-1,T=403K.α2苯乙醇的选择性均是先上升后下降.当压力为016MPa时,α2苯乙醇选择性为9214%.究其原因,一方面,低压有利于水相重整制氢反应的进行[31];另一方面,适当升高压力有利于苯乙酮加氢反应的进行[32].反应压力升高,反应产物氢分压也升高,促使水相重整反应的化学平衡逆向移动,氢气的生成速率下降[29];同时,苯乙酮加氢速率上升.因此,压力过高或过低,都不利于目标产物的生成.2.2.3 苯乙酮浓度的影响 表4为苯乙酮浓度对其加氢反应的影响.可以看出,随着浓度的增加,苯乙酮的转化率逐渐下降;α2苯乙醇的选择性则先升高后降低.在苯乙酮浓度为0143mol/L时,α2苯乙醇的选择性达到9314%;副产物α2环己基乙醇的选择性逐渐下降.当苯乙酮浓度为1119mol/L时,几乎没有α2环己基乙醇生成.这可能是苯乙酮在催化剂上吸附构型随覆盖度变化而引起的结果.在低覆盖度时,苯乙酮可能有以羰基为主和苯环为辅的两种表面吸附形式;在高覆盖度时,苯乙酮以苯环形式的吸附逐渐减少,直至完全以羰基的形式吸附在催化剂表面[33].表4 苯乙酮浓度对其加氢反应的影响Table4 Effect of concentration on hydrogenation of acetophenonec(PhCOMe)/(mol/L)X(PhCOMe)/%Product selectivity(w/%)PhCH(OH)Me PhEt C6H11CH(OH)Me C6H11Et 03364.388.610.4 1.00 0.4344.693.4 5.90.700.9240.490.49.10.501.1935.889.99.500.6Reaction conditions:n(H2O)/n(CH3OH)=4/3,L HSV=0171h-1,p(Ar)=0152MPa,T=403K.图1 液体空速对苯乙酮加氢反应的影响Fig1 Effect of L HSV on hydrogenation of acetophenone(1)X(PhCOMe),(2)S(PhCH(OH)Me),(3)S(PhEt),(4)Y(PhCH(OH)Me)(Reaction condition:n(PhCOMe):n(MeOH):n(H2O)=1∶15∶20,p(Ar)=0152MPa,T=403K.)2.2.4 空速的影响 考察了液体空速对苯乙酮加氢反应的影响,结果示于图1.可以看出,随着空速的增大,苯乙酮的转化率先急剧下降后缓慢下降;α2苯乙醇的选择性存在一个先急剧升高后缓慢降低的过程;乙苯的选择性则是先急剧下降后缓慢上升.空速为0171h-1时,α2苯乙醇的选择性达到9014%.这是由于从苯乙酮加氢到α2苯乙醇再到乙苯为串联反应,存在一个最佳反应时间.表5 水与甲醇的配比对苯乙酮加氢反应的影响Table5 Effect of H2O/MeOH molar ratio on hydrogenationof acetophenonen(H2O)n(MeOH)X(PhCOMe)%Product selectivity(w/%)PhCH(OH)Me PhEt C6H11CH(OH)Me3 51.392.6 4.9 2.5257.992.8 5.7 1.51.3366.495.1 4.00.9161.594.6 4.3 1.10.7554.892.6 6.80.6Reaction conditions:c(PhCOMe)=0143mol/L,L HSV=0171h-1,p(Ar)=016MPa,T=403K.2.2.5 原料配比的影响 原位加氢的氢来源于水相重整反应,不同的水与甲醇配比对甲醇水相重整制氢有重要影响,进而影响苯乙酮加氢反应性能.表5为水与甲醇的配比对苯乙酮加氢反应的影响.可以看出,水与甲醇存在一个最佳配比,当水/甲醇摩尔比为1133时,苯乙酮的转化率和α2苯乙醇的选择性均达到最高值.由482催 化 学 报第28卷于在不同配比下,水相重整制氢的产氢速率存在明显差异,且甲醇在催化剂表面会与苯乙酮发生竞争吸附.在这两个因素的共同作用下,造成过高或过低的甲醇浓度都不利于获得较高的苯乙酮转化率和α2苯乙醇选择性.2.3 偶合对甲醇水相重整反应的影响 苯乙酮在Raney Ni 上原位加氢包括甲醇水相重整制氢和苯乙酮原位加氢两步反应.图2为苯乙酮液相原位加氢的反应机理,吸附在催化剂活性中心上的氢来自甲醇水相重整反应.甲醇在Raney Ni催化剂作用下存在着C-H 键断裂(Ⅰ)和C-O 键断裂(Ⅱ)两个过程,其中C-H 键的断裂生成H 2和CO ,CO 进一步和水发生水汽转换反应生成H 2和CO 2;C-O 键的断裂则生成副产物CH 4,同时在Raney Ni 催化剂作用下的氢很容易与反应过程中产生的CO 和CO 2生成CH 4等副产物[27].苯乙酮有两个不饱和官能团(苯环和羰基),羰基的选择加氢对催化剂和氢的活性均有较高的要求.总的来说,羰基的诱导效应及共轭效应都具有吸电子能力,由此羰基的电子云密度较大促使它优先与吸附在活性金属表面的氢原子发生加成反应,羰基加氢的选择性相对较高.此外,目标产物α2苯乙醇的羟基是烯丙位的官能团,活性较高,发生氢解反应生成副产物乙苯是苯乙酮加氢反应选择性低的主要原因.本研究中也发现苯乙酮加氢过程的主要副产物是乙苯,只有少量的芳环加氢副产物.图2 苯乙酮液相原位加氢的反应机理Fig 2 Reaction mechanism for aqueous 2phase i n 2sit u hydrogenation of acetophenone(The asterisk represents a surface metal site.)表6 甲醇水相重整制氢及其与苯乙酮加氢的偶合反应体系中的气相实验数据Table 6 G aseous products for aqueous 2phase reforming of methanol coupled with hydrogenation of acetophenonen (H 2O )n (MeOH )p (Ar )MPa Production rate (μmol/(g ・min ))H 2H 32CH 4CH 34CO 2CO 32Selectivity (w /%)H 2H 323 0.42 5.56 1.640.400.28 2.00 2.5277.286.720.47 4.52 1.440.640.52 1.72 2.0863.873.31.30.52 2.440.840.960.32 1.12 1.6439.178.210.56 2.400.440.720.56 1.04 1.4445.462.70.80.602.040.480.360.640.801.1258.651.53After combination coupling. 对于醇类水相重整制氢反应而言,产生的氢原位应用于有机物的液相催化加氢反应,吸附在催化剂表面的活性氢原子被及时转移到有机物中,这可抑制烷基化副反应的发生,提高水相重整制氢反应氢气的选择性.表6为甲醇水相重整制氢及其与苯乙酮加氢的偶合反应体系中的气相实验数据.当甲醇水相重整反应与苯乙酮加氢还原反应偶合形成新的液相催化加氢体系后,甲醇水相重整制氢反应过582第3期姜 莉等:甲醇水相重整制氢原位还原苯乙酮制备α2苯乙醇程中氢气的选择性明显提高,同时气相中的总碳量也明显地提高.这说明甲醇水相重整制氢反应的转化率也得到了提高,并且没有检测到CO的存在.因此,通过两个反应的偶合,醇类水相重整制氢反应的活性和选择性均得到了进一步提高.3 结论 与传统的氢气还原法和异丙醇转移加氢还原法相比,利用甲醇水相重整制氢反应产生的氢原位还原苯乙酮可以得到更高的α2苯乙醇选择性.这可能是羰基高选择加氢(避免了氢解副反应的发生)的一种新的有效途径.同时,反应过程中水相重整产生的氢被及时从催化剂的活性中心位上移走,也明显提高了水相重整制氢反应的选择性.当然,“苯乙酮+甲醇+水”反应体系是一个由多步反应过程偶合成的总包反应体系,所以反应条件对总包反应的性能有明显的影响.在优化反应条件下,α2苯乙醇的选择性可达9511%.同时,由于原位加氢还原过程涉及多个反应,所以催化剂的进一步筛选对于甲醇水相重整制氢反应和苯乙酮加氢制备α2苯乙醇反应在质量上的匹配十分重要.参考文献1 Subbiah 5919991.19992 Y oshimoto H,Okawa K,Tamai Y.J P2000041655.20003 Rajashekharam M V,Bergault I,Fouilloux P,Schweich D,Delmas H,Chaudhari R V.Catal Today,1999,48(124):834 Lin S D,Sanders D K,Vannice M A.A ppl Catal A, 1994,113(1):595 Ohkuma T,Ooka H,Hashiguchi S,Ikariya T,Noyori R.J A m Chem Soc,1995,117(9):26756 Doucet H,Ohkuma T,Murata K,Y okozawa T,K ozawa M,K atayama E,England A F,Ikariya T,Noyori R.A ngew Chem,Int Ed,1998,37(12):17037 Noyori R,Hashiguchi S.Acc Chem Res,1997,30(2):97 8 Noyori R,Ohkuma T.A ngew Chem,Int Ed,2001,40(1):409 K ajitani M,Suzuki N,Abe T,K aneko Y,K asuya K, Takahashi K,Sugimori A.B ull Chem Soc Japan,1979, 52(8):234310 Masson J,Cividino P,Bonnier J M,Fouilloux P.S tud S urf Sci Catal,1991,59:24511 Bonnier J M,Damon J P,Masson J.A ppl Catal,1988, 42(2):28512 徐长青,朱大建,李光兴.化学试剂(Xu Ch Q,Zhu D J,Li G X.Chem Reag),2005,27(3):12913 徐长青,朱大建,李光兴.分子催化(Xu Ch Q,Zhu D J,Li G X.J Mol Catal(China)),2004,18(4):281 14 Chen C2S,Chen H2W,Cheng W2H.A ppl Catal A, 2003,248(1/2):11715 Santori G F,Moglioni A G,Vetere V,Iglesias G Y M, Casella M L,Ferretti O A.A ppl Catal A,2004,269(1/2):21516 Malyala R V,Rode C V,Arai M,Hegde S G,Chaudhari R V.A ppl Catal A,2000,193(1/2):7117 Casagrande M,Storaro L,Tolon A,Lenarda M,Frattini R,Rodriguez2Castellon E,Maireles2Torres P.J Mol Catal A,2002,188(1/2):13318 Mathew S P,Rajasekharam M V,Chaudhari R V.Catal Today,1999,49(123):4919 Ikenaga T,Matsushita K,Shinozawa J,Y ada S,Takagi Y.Tet rahedron,2005,61(8):210520 Zaccheria F,Ravasio N,Psaro R,Fusi A.Tet rahedron L ett,2005,46(21):369521 Drelinkiewicza A,Waksmundzka A,Makowski W, S obczak J W,Krol A,Z ieba A.Catal L ett,2004,94(3/4):14322 Masson J,Vidal S,Cividino P,Fouilloux P,Court J.A ppl Catal A,1993,99(2):14723 Masson J,Cividino P,Court J.A ppl Catal A,1997, 161(1/2):19124 Bergault I,Fouilloux P,Joly2Vuillemin C,Delmas H.J Catal,1998,175(2):32825 Hamar2Thibault S,Masson J,Fouilloux P,Court J.A p2 pl Catal A,1993,99(2):13126 Shabaker J W,Huber G W,Dumesic J A.J Catal, 2004,222(1):18027 Cortright R D,Davda R R,Dumesic J A.N ature,2002, 418(6908):6428 Huber G W,Shabaker J W,Dumesic J A.Science, 2003,300(5628):207529 白赢,卢春山,马磊,陈萍,郑遗凡,李小年.催化学报(Bai Y,Lu Ch Sh,Ma L,Chen P,Zheng Y F,Li X N.Chin J Catal),2006,27(3):27530 李小年,项益智.中国科学(Li X N,Xiang Y Zh.Sci2 ence in China),In press31 K im W B,Voitl T,Rodriguez2Rivera G J,Evans S T, Dumesic J A.A ngew Chem,Int Ed,2005,44(5):778 32 黄艳轶,马红霞,熊伟,陈华,李贤均.催化学报(Huang Y Y,Ma H X,Xiong W,Chen H,Li X J.Chin J Catal),2004,25(12):96233 王友臻.[硕士学位论文].上海:复旦大学(Wang Y Zh.[MS Dissertation].Shanghai:Fudan Univ),2004(Ed W GZh)682催 化 学 报第28卷。