Intrinsic nanoscale phase separation of bulk As2S3 glass
基于金刚石氮–空位色心的微波磁场成像技术的可靠性研究

Vol. 40, No. 6航 天 器 环 境 工 程第 40 卷第 6 期682SPACECRAFT ENVIRONMENT ENGINEERING2023 年 12 月https:// E-mail: ***************Tel: (010)68116407, 68116408, 68116544基于金刚石氮–空位色心的微波磁场成像技术的可靠性研究唐雨桐1,叶 安1,付鼎元1,李晓林1,张 超2*(1. 华东理工大学 物理学院,上海 200237; 2. 北京卫星环境工程研究所,北京 100094)摘要:相对于单片微波集成电路(MMIC)芯片的设计与制造工艺发展,芯片的测试与失效分析研究进展缓慢。
文章首先调研了基于金刚石氮–空位(NV)色心的高空间分辨率微波磁成像应用及通过磁成像技术反演电流分布的技术进展;继而进行了基于NV色心系综微波磁场成像技术的MMIC热态可靠性研究。
结果表明:利用基于金刚石NV色心的微波磁场成像技术,对毫米波微波芯片表面的二维矢量场进行高空间分辨率、高灵敏度的快速成像与重构,可以采集芯片在正常和非正常工作状态下的磁场成像信息;进一步对微波芯片内部的信息进行反演重建,可以实现芯片内部故障点的精确定位诊断和潜在故障点排除。
所做研究有望为芯片设计、生产、测试提供可靠性诊断。
关键词:单片微波集成电路;失效模式分析;磁成像;氮−空位色心;芯片热态可靠性中图分类号:TN707; V443文献标志码:A文章编号:1673-1379(2023)06-0682-10 DOI: 10.12126/see.2023043Study on the reliability of microwave magnetic field imaging technique based ondiamond nitrogen-vacancy color centersTANG Yutong1, YE An1, FU Dingyuan1, LI Xiaolin1, ZHANG Chao2*(1. School of Physics, East China University of Science and Technology, Shanghai 200237, China;2. Beijing Institute of Spacecraft Environment Engineering, Beijing 100094, China)Abstract: Compared with the development of chip design and manufacturing of monolithic microwave integrated circuits (MMIC), the research on testing and failure analysis of chips has been progressing slowly. In this paper, the application of high-spatial resolution microwave magnetic imaging based on diamond nitrogen-vacancy (NV) color center and the technical progress of inversion current distribution by magnetic imaging were firstly reviewed. On this basis, the thermal reliability of MMIC based on NV color center ensemble microwave magnetic field imaging technology was studied. The results show that, by using the microwave magnetic field imaging technology based on the diamond NV color center, through the rapid imaging and reconstruction of the two-dimensional vector field on the surface of the millimeter wave microwave chip with high spatial resolution and high sensitivity, the magnetic field imaging information of both normal and abnormal working states of chips can be collected. The precise positioning and diagnosis of the fault points inside the chip and the elimination of potential fault points can be realized by further inversion and reconstruction of the internal information of the microwave chip. The proposed research is expected to provide reliability diagnosis for chip design, production and testing.Keywords: monolithic microwave integrated circuit; failure mode analysis; magnetic imaging; nitrogen-vacancy color center; chip thermal reliability收稿日期:2023-04-06;修回日期:2023-12-01基金项目:民用航天预研项目D040301引用格式:唐雨桐, 叶安, 付鼎元, 等. 基于金刚石氮–空位色心的微波磁场成像技术的可靠性研究[J]. 航天器环境工程, 2023, 40(6): 682-691TANG Y T, YE A, FU D Y, et al. Study on the reliability of microwave magnetic field imaging technique based on diamond nitrogen-vacancy color centers[J]. Spacecraft Environment Engineering, 2023, 40(6): 682-6910 引言自20世纪60年代以来,以微带线[1]为代表的微波与毫米波混合集成电路(microwave integrated circuits, MIC)以其结构紧凑、体积小、重量轻、造价低以及便于同有源器件相连等优点而得到迅速发展;继而随着新型集成介质传输线、介质波导以及谐振器/谐振腔在MIC的使用[2]以及MIC加工工艺的进一步成熟,出现了将大量有源器件和无源器件/组件或模块集成于一块集成电路(integrated circuit, IC)[3]的单片微波集成电路(monolithic microwave integrated circuit, MMIC)[4]。
一种聚乙二醇修饰的重组人粒细胞集落刺激因子及其制备方法[发明专利]
![一种聚乙二醇修饰的重组人粒细胞集落刺激因子及其制备方法[发明专利]](https://img.taocdn.com/s3/m/afcc32df376baf1ffc4faded.png)
专利名称:一种聚乙二醇修饰的重组人粒细胞集落刺激因子及其制备方法
专利类型:发明专利
发明人:徐明波,杨仲凡,连治国,王俊玲,许可,刘迎,梁果义,吴彦卓
申请号:CN200810223549.1
申请日:20081008
公开号:CN101711876A
公开日:
20100526
专利内容由知识产权出版社提供
摘要:本发明公开了一种聚乙二醇修饰重组人粒细胞集落刺激因子偶联物及其制备方法,具体地涉及一种聚乙二醇定点修饰重组人粒细胞集落刺激因子N端脯氨酸的偶联物,此物质是该技术领域中所没有的,与国外已上市同类产品相比,该物质具有更低的免疫原性,更少的活性损失和更高的制备收率。
申请人:北京双鹭药业股份有限公司
地址:100041 北京市石景山区八大处高科技园区中园路9号
国籍:CN
更多信息请下载全文后查看。
固体核磁分峰拟合

固体核磁分峰拟合1.引言1.1 概述在固体核磁共振(NMR)技术中,分峰拟合是一种常用的分析方法。
当我们对某个固体样品进行核磁共振实验时,通过强磁场作用下,样品原子核会产生特定的共振信号。
这些信号可以被记录并转换为核磁共振谱图,其中包含了丰富的信息。
然而,由于固体样品中原子核的环境复杂,谱图往往呈现多个峰的形式。
为了从谱图中准确提取出各个峰的参数,我们需要借助分峰拟合方法。
分峰拟合可以将谱图中的峰拟合为高斯曲线或者洛伦兹曲线,从而得到每个峰的位置、面积、峰宽等信息。
分峰拟合在固体核磁共振分析中有着广泛的应用。
它可以用于定量分析样品中某个特定分子的含量,还可以帮助我们研究样品的结构和动力学性质。
通过分峰拟合,我们可以得到更加精确和详细的核磁共振谱图信息,为进一步的研究提供重要的基础。
本文将介绍固体核磁共振技术以及核磁共振谱图的分析方法,并重点探讨了分峰拟合的原理和应用。
通过对分峰拟合方法的研究和应用,我们可以更准确地获得样品的核磁共振谱图信息,并为相关领域的研究提供有力支持。
1.2文章结构文章结构部分的内容如下:1.2 文章结构本文主要分为三个部分进行讨论。
首先,在引言部分将对固体核磁分峰拟合的概述进行介绍,包括该技术的基本原理、应用领域以及研究意义。
其次,在正文部分,将详细介绍核磁共振技术的基本知识,包括核磁共振的原理、仪器设备和实验操作方法等内容。
同时,还将探讨核磁共振谱图的分析方法,包括化学位移、耦合常数和峰形分析等方面内容。
最后,在结论部分将详细介绍固体核磁分峰拟合的方法,并讨论其在实际应用中的前景和潜在的发展方向,以及可能的挑战和限制。
通过这样的结构安排,本文旨在给读者提供一个全面而深入的了解固体核磁分峰拟合技术的文章。
1.3 目的本文的目的是探讨固体核磁共振谱图的分峰拟合方法及其在实际应用中的前景。
通过对固体样品进行核磁共振谱图分析,我们可以获取关于样品分子结构、动力学信息以及相互作用的重要信息。
基于金纳米粒子局域表面等离子体共振吸收检测卡托普利
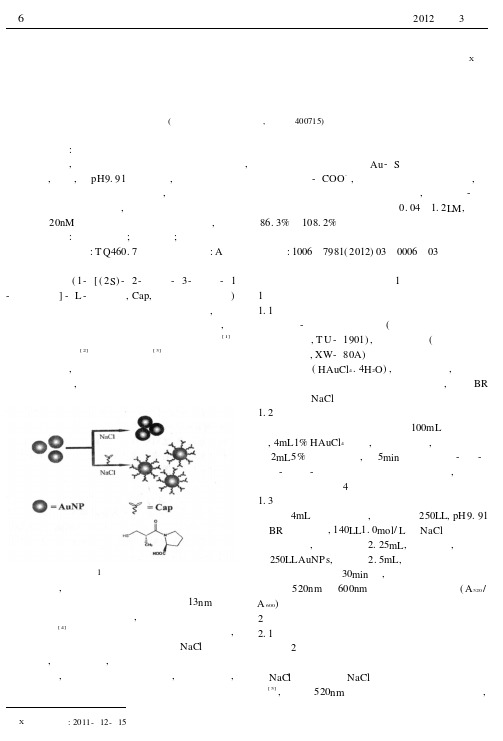
基于金纳米粒子局域表面等离子体共振吸收检测卡托普利X许 丹(西南大学化学化工学院,重庆 400715) 摘 要:柠檬酸根稳定的金胶在一定盐浓度下由于盐的电荷屏蔽效应而发生聚集。
加入一定浓度的卡托普利后,由于卡托普利分子中含有巯基和羧基,其分子中的巯基可以通过Au -S 键连在金纳米粒子表面,同时,在pH9.91的条件下,其分子中的羧基去质子化形成-COO -,导致金胶表面负电荷增多,纳米粒子之间的静电排斥力增大,金胶的聚集得到了抑制。
基于金胶由聚集到分散的现象,利用紫外-可见吸收光谱进行表征,建立了定量检测卡托普利含量的方法。
该方法的线性范围为0.04~1.2L M ,检出限为20nM 。
将此方法用于合成样的检测,回收率在86.3%~108.2%之间。
关键词:金纳米粒子;卡托普利;表面等离子体共振吸收 中图分类号:T Q460.7 文献标识码:A 文章编号:1006—7981(2012)03—0006—03 卡托普利(1-[(2S )-2-甲基-3-巯基-1-氧化丙基]-L -脯氨酸,Cap,结构如下图所示)是一种人工合成的血管紧张素转换酶抑制剂,目前广泛应用于治疗高血压及心力衰竭等疾病。
目前,定量测定卡托普利的分析方法有高效液相色谱法[1]、氧化还原滴定法[2]及化学发光法[3]等。
这些方法虽然灵敏度较高,但存在一些缺点如仪器设备昂贵、操作繁琐等。
因此,建立简便、快速、灵敏度高的检测卡托普利的方法仍然具有十分重要的意义。
图1 实验原理图近年来,金纳米粒子由于其独特的表面等离子体共振吸收性质被广泛用于色度传感。
13nm 柠檬酸根包被的金胶呈现酒红色,一旦发生聚集则呈现紫色或蓝色[4]。
这种颜色的改变很容易用肉眼捕获,不需要复杂的仪器。
金胶在一定浓度的NaCl 溶液中发生聚集,在本研究中,我们发现当体系中同时存在卡托普利后,金胶的聚集能得到抑制,基于此现象,建立了一种金胶由聚集到分散的状态来高灵敏检测卡托普利的新方法。
N个热囚禁离子的GHZ态的制备(英文)

N个热囚禁离子的GHZ态的制备(英文)
黎三华;郑小娟
【期刊名称】《量子光学学报》
【年(卷),期】2008(14)2
【摘要】我们提出了一个简单的方法产生N个囚禁离子的GHZ态。
在这个方法中,初始时处于相同态的N个两能级离子被囚禁在一个线性阱中,同时被两束均匀的经典激光照射。
这个方法对振动模的加热不敏感,从退相干的观点来看这点很重要。
【总页数】4页(P126-129)
【关键词】量子纠缠;GHZ态;囚禁离子
【作者】黎三华;郑小娟
【作者单位】中南大学物理科学与技术学院
【正文语种】中文
【中图分类】O431
【相关文献】
1.多个囚禁离子未知类电子GHZ态的确定量子隐形传送 [J], 郑小娟;廖湘萍
2.3个热囚禁离子GHZ态的制备 [J], 郑小娟;方卯发
3.一个囚禁离子运动压缩数态的制备(英文) [J], 郑仕标
4.囚禁离子多体纠缠态的简单制备方案(英文) [J], 钟锋;于立志;熊文元
5.在囚禁离子系统制备三离子树型三维纠缠态 [J], 林秀;杨榕灿
因版权原因,仅展示原文概要,查看原文内容请购买。
英文-无机纳米材料光解水ppt课件
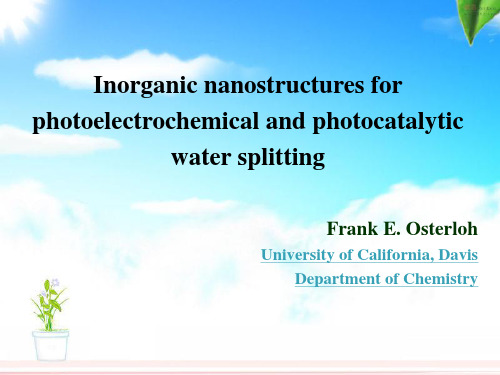
Contents
1
Introduction
2
Brief history
3 Inorganic nanostructures
4 Conclusion and outlook
carbon free energy technology
The solar energy received on the Earth’s surface meets current and future human energy demand.
photoelectrochemical water splitting
Exploit scaling laws and specific effects at 3 the nanoscale to enhance the efficiency of
existing semiconductors and metal oxides
three main strategies
Coat conventional photovoltaic cells with 1 cocatalysts for water splitting or with
protecting layers to inhibit photocorrosion
Development of new metal oxide materials 2 that combine suitable properties for
Advantages
✓ Shortened carrier collection pathways ✓ Improved light distribution ✓ Quantum size confinement ✓ Potential determining ions (PDI) ✓ Surface area-enhanced charge transfer ✓ Multiple exciton generation
无机水合盐相变材料Na_2SO_4_10H_2O的研究进展_黄金
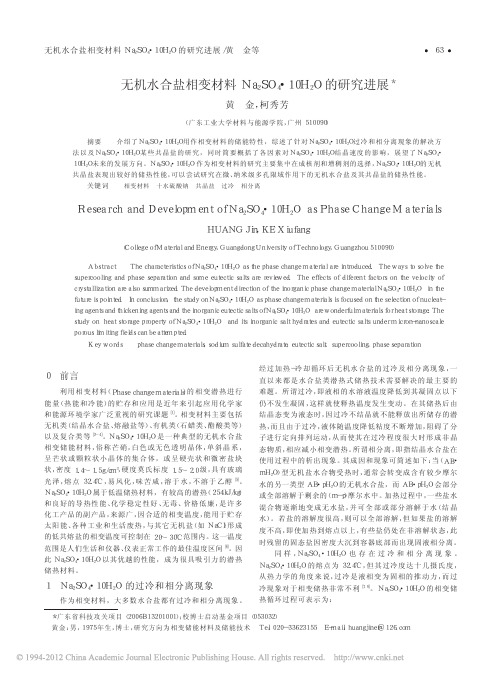
作为相变材料, 大多数水合盐都有过冷和相分离现象。
经过加热- 冷却循环后无机水合盐的过冷及相分离现象, 一 直以来都是水合盐类潜热式储热技术需要解决的最主要的 难题。所谓过冷, 即液相的水溶液温度降低到其凝固点以下 仍不发生凝固, 这样就使释热温度发生变动。在其储热后由 结晶态变为液态时, 因过冷不结晶就不能释放出所储存的潜 热, 而且由于过冷, 液体随温度降低粘度不断增加, 阻碍了分 子进行定向排列运动, 从而使其在过冷程度很大时形成非晶 态物质, 相应减小相变潜热。所谓相分离, 即指结晶水合盐在 使用过程中的析出现象。其成因和现象可简述如下: 当( AB· mH2O) 型无机盐水合物受热时, 通常会转变成含有较少摩尔 水的另一类型 AB·pH2O 的无机水合盐, 而 AB·pH2O 会部分 或全部溶解于剩余的( m- p) 摩尔水中。加热过程中, 一些盐水 混合物逐渐地变成无水盐, 并 可 全 部 或 部 分 溶 解 于水( 结 晶 水) 。若盐的溶解度很高, 则可以全部溶解, 但如果盐的溶解 度不高, 即使加热到熔点以上, 有些盐仍处在非溶解状态, 此 时残留的固态盐因密度大沉到容器底部而出现固液相分离。
Key wor ds phase change materials, sodium sulfate decahydrate, eutectic salt, supercooling, phase separation
0 前言
利用相变材料( Phase change materials) 的 相 变 潜 热进 行 能量( 热能和冷能) 的贮存和应用是近年来引起应用化学 家 和 能 源 环 境 学 家 广 泛 重 视 的 研 究 课 题 [ 1] 。相 变 材 料 主 要 包 括 无机类( 结晶水合盐、熔融盐等) 、有机类( 石蜡类、酯酸类等) 以及复合类等[2~4]。Na2SO4·10H2O 是一种典型的无机水合盐 相变储能材料, 俗称芒硝, 白色或无色透明晶体, 单斜晶系, 呈芒状或颗粒状小晶体的集合体, 或呈硬壳状和微密盐块 状, 密度 1.4~1.5g/cm3, 硬度莫氏标度 1.5~2.0 级, 具有玻璃 光 泽 , 熔 点 32.4℃, 易 风 化 , 味 苦 咸 , 溶 于 水 , 不 溶 于 乙 醇 [5]。 Na2SO4·10H2O 属于低温储热材料, 有较高的潜热( 254kJ/kg) 和良好的导热性能、化学稳定性好、无毒、价格低廉, 是许多 化工产品的副产品, 来源广, 因合适的相变温度, 能用于贮存 太阳能、各种工业和生活废热, 与其它无机盐( 如 NaCl) 形成 的低共熔盐的相变温度可控制在 20~30℃范围内。这一温度 范围是人们生活和仪器、仪表正常工作的最佳温度区间[6]。因 此 Na2SO4·10H2O 以其优越的性能, 成为很具吸引力 的 潜 热 储热材料。
InN p-i-n Nanowire Solar Cells on Si

InN p-i-n Nanowire Solar Cells on Si Hieu Pham Trung Nguyen,Yi-Lu Chang,Ishiang Shih,and Zetian Mi(Invited Paper)Abstract—In this paper,we report thefirst experimental demon-stration of InN nanowire solar cells.By employing an in situ de-posited In seeding layer,we have achieved electronically pure, nearly intrinsic InN nanowires directly on Si(111)substrates by molecular beam epitaxy.The growth and characterization of Si-and Mg-doped InN nanowires is also investigated,which can ex-hibit superior structural and optical properties.We have further studied the epitaxial growth,fabrication,and characterization of InN:Si/i-InN and InN:Mg/i-InN/InN:Si axial nanowire structures on p-type and n-type Si(111)substrates,respectively.With the use of a CdS surface passivation,InN:Mg/i-InN/InN:Si nanowire homojunction solar cells exhibit a promising short-circuit cur-rent density of∼14.4mA/cm2and power-conversion efficiency of∼0.68%under simulated one-sun(AM1.5G)illumination.This work suggests thefirst successful demonstration of p-type doping in InN nanowires and also constitutes important progress for the development of InGaN-based,full-solar-spectrum photovoltaics.Index Terms—Nanotechnology,optoelectronic devices,p-i-n diodes,solar cells.I.I NTRODUCTIONS INCE the recent discovery of InN bandgap at∼0.6to0.7eV,the use of InN and related alloys for solar-cell appli-cations has been proposed and intensively investigated[1]–[9]. InN also exhibits several important attributes,including a rel-atively high absorption coefficient,high carrier mobility,and large drift velocity that are required for high-efficiency pho-tovoltaics.An energy-conversion efficiency of over20%is ex-pected for an ideal InN single-junction solar cell[10].Addition-ally,InN may be integrated with Si or other thinfilms to form heterojunction solar cells or be incorporated as a critical subcell for future InGaN-based full-solar-spectrum multijunction de-vices[11].However,InN thinfilms generally exhibit extremely poor quality,due to the lack of suitable substrates,and,to date, an InN solar cell has not been demonstrated.It is therefore imperative to explore InN nanostructures,including nanowires and quantum dots,which can exhibit drastically reduced dis-location densities,owning to the highly effective lateral stress relaxation.Additional advantages offered by the1-D nanowires for solar-cell applications include a direct path for carrier trans-port,an increased surface area for enhanced light absorption,Manuscript received July13,2010;revised August10,2010;accepted August10,2010.Date of publication November11,2010;date of current ver-sion August5,2011.This work was supported in part by the Natural Sciences and Engineering Research Council of Canada and in part by the Hydro-Quebec Nano-Engineering Scholar Program at McGill University.The authors are with the Department of Electrical and Computer Engi-neering,McGill University,Montreal,QC H3A2A7,Canada(e-mail:zetian. mi@mcgill.ca).Digital Object Identifier10.1109/JSTQE.2010.2082505and the compatibility with Si or other low-cost,large-area sub-strates[12]–[14].The growth and characterization of InN nanowires have been intensively studied.InN nanowires can be formed using the vapor–liquid–solid growth process,the spontaneous formation under nitrogen-rich conditions,or the selective-area growth on nano-patterned substrates[15]–[17].Well-spaced,vertically aligned InN nanowires have been grown on Si and other sub-strates using plasma-assisted molecular beam epitaxy(MBE) or metal–organic chemical vapor deposition.The resulting InN nanowires generally exhibit a wurtzite crystal structure,with the growth direction oriented along the c-axis.However,due to the very low dissociation temperature of InN and the very high surface migration rate of In,conventional growth techniques yield InN nanowires with tapered surface morphology and large stacking fault and dislocation densities[18]–[20],which de-crease the carrier diffusion length and severely limit the solar cell efficiency.The wires also exhibit very large size dispersion, with significant variations in the diameters and heights.Addi-tionally,due to the extremely low conduction band minimum of InN,any defects,dislocations or impurities generally form donors,thereby leading to very high electron densities,which are commonly measured in the range of∼1×1018cm−3,or higher for nominally nondoped InN[20]–[25].The resulting n-type degenerate InN nanowires exhibit poor optical properties, characterized by nearly temperature and power invariant pho-toluminescence emission spectra and photoluminescence peak energies(∼0.7to0.8eV)considerably larger than the bandgap of InN[20],[21],[26].The uncontrolled structural,electrical and optical properties have posed a significant challenge for the rational design and fabrication of InN nanowire solar cells. The realization of InN nanowire solar cells,as well as many other semiconductor devices,has been further limited by the difficulty in achieving p-type doping.In this regard,Mg-doped InNfilms and the formation of p-type carriers have been in-vestigated both theoretically and experimentally[27]–[30].A Fermi level shift toward the valence band was measured in InN:Mg layers[30],and the possibility of p-type doping is further suggested by electrolyte capacitance-voltage measure-ments[28],[31].Free-to-acceptor photoluminescence emission has also been observed in Mg-doped InN,with an activation energy of∼61meV derived for the Mg acceptor[29].In order to compensate the presence of large electron densities of nom-inally nondoped InN as well as the associated surface electron accumulation,a relatively high concentration of Mg dopant is required,which,however,may lead to the formation Mg-related, donorlike defects[29],[32],[33].Additionally,the growth and characterization of InN:Mg nanowires,to the best of our knowl-edge,has not been reported.It has been observed that the1077-260X/$26.00©2010IEEEincorporation of Mg can significantly affect the formation and structural properties of GaN nanowires[34],[35].The presence of Mg can greatly reduce the nanowire nucleation time and en-hance the growth rate on the nonpolar surfaces,thereby leading to wires with increased diameters and reduced lengths.Deterio-rated crystal structures were further observed at relatively high Mg concentrations.It is therefore of tremendous importance to develop nearly intrinsic InN nanowires as well as InN nanowire p-n junctions, in order to exploit the full potential of InN for third generation photovoltaics.In this context,we have performed a detailed in-vestigation of the MBE growth and characterization of nearly intrinsic and Si-and Mg-doped InN nanowires on Si(111) substrates without any external metal catalyst.Under optimized growth conditions,the wires exhibit nontapered surface mor-phology and excellent structural and optical properties.An ex-tremely narrow spectral linewidth of∼8meV,compared to the commonly reported values of50–100meV for n-type degener-ate InN nanowires,have been achieved for nearly intrinsic InN nanowires.Effects of Si and Mg incorporation on the structural and optical properties of InN nanowires have also been investi-gated.We have further studied the epitaxial growth,fabrication, and characterization of InN:Si/i-InN and InN:Mg/i-InN/InN:Si nanowire axial structures on p-type and n-type Si(111)sub-strates,respectively.With the use of CdS surface passivation, InN:Mg/i-InN/InN:Si nanowire homojunction solar cells exhibit a promising short-circuit current density of∼14.4mA/cm2and power-conversion efficiency of∼0.68%under simulated one-sun(AM1.5G)illumination.In Section II,the MBE growth and characterization of nearly intrinsic and Si-and Mg-doped InN nanowires isfirst presented.In Section III,we describe the design and fabrication of InN nanowire solar cells monolith-ically grown on Si.The characterization results and analysis are presented in Section IV.Finally,conclusions are made in Section V.II.MBE G ROWTH AND C HARACTERIZATIONOF I N N N ANOWIRESA.Nearly Intrinsic InN Nanowires on Si Substrates Electronically pure InN nanowires were grown on Si(111) substrates by plasma-assisted MBE under nitrogen-rich con-ditions without any external metal catalyst.To achieve high-quality InN nanowires with controllable structural properties, we investigated the self-catalytic growth of InN nanowires.In this process,a thin(∼0.5to1.6nm)In seeding layer isfirst deposited on the Si substrate surface prior to growth initia-tion[36],[37].The thin In layer forms nanoscale liquid droplets at elevated temperatures,which provides well-defined nucle-ation centers for the formation and growth of InN nanowires. Subsequently,the InN nanowire growth is carried out at a nom-inal growth rate of0.6˚A/s,nitrogenflow rate of1.0–2.0sccm, growth temperature of440–520◦C,and RF plasma forward power of∼400W.The scanning electron micrographs of InN nanowires grown on Si without and with the use of In seeding layer are shown in Fig.1(a)and(b),respectively.It is evident that significantly improved structural properties,with a nontapered surfacemor-Fig.1.Scanning electron micrographs of nondoped InN nanowires grown on Si(111)substrates by MBE(a)without and(b)with the use of an in situ deposited In seeding layer.phology and nearly identical heights,can be obtained by using an in situ deposited In seeding layer.Detailed analysis,described elsewhere[36],further confirms that the wires have a wurtzite crystal structure,orient along the c-axis and are relatively free of dislocations.Important for practical device applications is a precise control of the carrier concentration and conductivity of InN nanowires. The residual electron density of InN can be derived from a Hall-effect measurement or by analyzing the photoluminescence spectral linewidth measured at low temperatures[38],[39].For conventional n-type degenerate InN,the measured photolumi-nescence linewidths are generally in the range of50–100meV, which correspond to residual electron densities of∼1018cm−3, or higher[21].Illustrated in Fig.2are the photoluminescence spectra for the presently achieved nontapered InN nanowires measured under various laser powers at5K.An extremely narrow spectral linewidth of8meV was obtained under low excitation conditions.Detailed analysis revealed that the elec-tron density in the undoped InN nanowires is∼2×1015cm−3, or less,which is nearly a factor of500times smaller than the commonly reported values[20]–[25],suggesting,for thefirst time,the achievement of nearly intrinsic InN.The presence of an extremely low level of carrier concentration in the undoped InN nanowires is also directly reflected in the power-dependent photoluminescence emission,as shown in Fig.2.With increas-ing excitation power,there is a considerable blueshift in the photoluminescence peak energy,accompanied by a significantFig.2.Photoluminescence spectra of nondoped InN nanowires on Si(111)measured at 5K under various laserpowers.Fig.3.Photoluminescence spectra of InN nanowires on Si(111)substrates for Si doping concentrations of ∼1×1018cm −3(dashed and dotted line),2×1017cm −3(dotted line),5×1016cm −3(dashed line),nondoped (solid line),measured at 5K.broadening of the spectral linewidth.Such a clear band-filling effect has not been previously observed in the photolumines-cence emission of n-type degenerate InN.B.Si and Mg-Doped InN NanowiresWith the achievement of nearly intrinsic InN nanowires,we have subsequently investigated the growth and optical proper-ties of Si-and Mg-doped InN nanowires.These nanowires are grown by introducing the respective dopants during nanowire growth without any modifications to the previously described growth conditions.Due to the significantly enhanced In adatom surface migration and the preferred growth along the nanowire length direction,the resulting doping level is generally smaller,compared to that of planar heterostructures.It is expected that the local dopant fluctuation in the wires may also be negligible,due to the relatively large (>100nm)wire diameters.For the Si doping concentrations considered (<∼1×1018cm −3),no morphological changes to the InN nanowires were observed.However,the incorporation of Si dopant can significantly mod-ify the optical properties of InN nanowires.Illustrated in Fig.3are the photoluminescence spectra of InN:Si nanowires measured at 5K for various Si doping lev-els.It is seen that,with increasing Si doping concentration,InN nanowires exhibit a considerable blueshift in the photolumines-cence peak energy,a drastic increase in the spectral linewidth,and a significant decrease in the luminescence efficiency.SuchFig.4.Scanning electron microscopy image of InN nanowires grown on Si(111)substrates with a relatively high Mg doping concentration.The wires show deteriorated structural properties,with the presence of slightly tapered surface morphology.effects have also been observed at elevated temperatures and under various laser powers.The observed Burstein–Moss shift,i.e.,the significant increase in the photoluminescence peak en-ergy and broadening of the spectral linewidth,can be well ex-plained by the increased electron densities with increasing Si doping concentrations.However,the exact origin of the drasti-cally reduced luminescence efficiency with increasing Si doping concentration remains unclear.One possible explanation is the increasing surface electron accumulation,induced by the bulk electron density that leads to a significantly increased spatial separation of charge carriers in the wires.As a consequence,the radiative efficiency is reduced with increasing Si doping concentration.The correlation between the surface charge ac-cumulation and the bulk electron density has been suggested by recent studies [22].Detailed discussions on the surface charge properties of nearly intrinsic and Si-doped InN nanowires are described elsewhere.From photoluminescence measurements of InN:Mg films,it was determined that the Mg acceptor activation energy was about 61meV [29].However,to the best of our knowledge,the growth and properties of InN:Mg nanowires have not been reported.In this study,InN:Mg nanowires,with Mg effusion cell temperatures varying from 185◦C to 235◦C,are grown and characterized.It was observed that,for relatively low Mg concentrations,InN nanowires with excellent surface morphol-ogy and structural properties can be obtained.However,with increasing Mg flux,the wires show increasing diameter and re-ducing length,potentially due to the reduced adatom surface migration.Similar effects have also been observed for GaN:Mg nanowires [34],[35].A further increase of the Mg concentration generally leads to a tapered surface morphology,as shown in Fig.4,and the generation of dislocations.Illustrated in Fig.5are the photoluminescence spectra of InN:Mg nanowires measured at 5K for various Mg effu-sion cell temperatures.It is observed that the photolumines-cence peak intensity decreases considerably with increasing MgFig.5.5K photoluminescence spectra of InN:Mg nanowires grown at differ-ent Mg effusion cell temperatures.incorporation,which may be directly related to the formation of Mg-related defects.A detailed investigation of the underly-ing mechanism as well as the electrical transport properties and p-type conductivity of InN:Mg wires will be published in the future.III.D ESIGN AND F ABRICATION OF I N NN ANOWIRE S OLAR C ELLS ON S IThree InN nanowire solar cell designs,schematically illus-trated in Fig.6(a)–(c),have been investigated.In thefirst ap-proach,as shown in Fig.6(a),the InN nanowire solar cells consist of∼0.3μm nondoped and0.4μm Si-doped sections, which are grown directly on p-type Si(111)substrates.Such a design does not require the use of p-type InN nanowires,which had not been demonstrated prior to this study.The second and third designs employ InN p-i-n axial homojunctions,illustrated in Fig.6(b)and(c),which comprise of∼0.8μm InN:Si,0.2μm nondoped InN,and0.3μm InN:Mg sections grown on n-type Si(111)pared to thefirst design,the p-n junc-tion is formed within the wires.It may be noted that there is a small variation in the thicknesses of the device intrinsic regions, which may not have any major impact on the device efficiency. One of the primary limitations for semiconductor nanowire devices is the significant nonradiative carrier recombination as-sociated with the presence of surface states,which can be greatly minimized by using core–shell or dot/well-in-a-wire nanoscale heterostructures[40],[41].In this regard,we have further inves-tigated the use of a CdS passivation technique in the third design. Illustrated in Fig.6(c),a thin(∼10nm)CdS layer is coated on the nanowire surface using a chemical bath deposition method at∼70◦C,wherein the bath consists of CdCl2,NH4Cl,and NH4OH,with a molecular proportion of2:20:20:200[42].The resulting CdS layer is nearly intrinsic and exhibits a very high resistivity(∼106Ω·cm)[43].Such a passivation/buffer layer has been widely used in the fabrication of CuIn(Ga)Se2solar cells and has led to the most efficient(∼19.9%)thinfilm solar cells ever reported[44].Growth conditions for the various nanowire samples have been carefully controlled to achieve high-quality InN nanowires with relatively large diameters(>150nm)to effectively enhance the light absorption[45].The fabrication process for InN nanowire solar cells on Si is briefly described for the second design.A polyimide(PI)resist Fig.6.Schematic illustrations of(a)InN:Si/i-InN nanowire solar cells grown on p-type Si substrates,(b)InN:Mg/i-InN/InN:Si nanowire solar cells grown on n-type Si substrates,and(c)CdS-passivated InN:Mg/i-InN/InN:Si nanowire solar cells grown on n-type Si substrates.The top contact consists of thin (∼15nm)Ni/Au or Ti/Au layers.layer isfirst spin-coated to fully cover the InN nanowires for surface planarization.The PI layer is subsequently etched using O2:CF4(1:4)dry-etching until the top region of the wires is exposed,as shown in Fig.7(a).The sample is thenflood-exposed with UV light and hard-baked at350◦C for∼30min to cure the PI.The top exposed section of the wires is patterned,using standard photolithography,into cells of various sizes(0.09–1.00mm2)and,a thin Ni/Au(5nm/10nm)p-metal contact is deposited on the cell surface,as shown in Fig.7(b),which is followed by the deposition of thick Ni/Au metal contact layers at the periphery of the devices.Ti/Au(30nm/150nm)layers are then deposited on the backside of the n-Si substrate as the n-metal contact.The sample is annealed at300–400◦C for60s.IV.R ESULTS AND D ISCUSSSIONSThe performance characteristics of InN nanowire solar cells on Si is measured under dark and illuminated(one sun at AM 1.5G)conditions.Characteristics of thefirst design,i.e.,the InN:Si/i-InN/p-Si heterojunction nanowire solar cells isfirstFig.7.45◦scanning electron microscopy images of(a)the InN nanowire ensemble spin-coated with a PI layer,with the top region of the wires exposed by dry etching and(b)the PI immersed wire ensemble after the deposition of Ni/Au contact layers.described.The current–voltage(I–V)response of such devices measured under dark conditions is shown in Fig.8(a).It is seen that InN/Si nanowire heterojunction design exhibits very poor diode characteristics,with rectifying ratios in the range of∼2 to4measured at−0.5and0.5V.At−1V,a leakage current of more than100mA/cm2was measured.As a consequence,no significant photo response was observed.The measured short-circuit current density and energy-conversion efficiency are less than2mA/cm2and0.01%,respectively.The underlying mech-anism for the extremely poor device performance has been in-vestigated.Due to the very large electron affinity(∼5.8eV) of InN,its conduction band minimum is positioned well below the valence band maximum of Si.In addition,a thin(∼2to 3nm)amorphous layer(SiN x)is generally formed at the InN/Si misfit interface[36].The resulting energy-band diagram of the n-InN/i-InN/p-Si heterostructure under thermal equilibrium is schematically shown in Fig.8(b).The depletion occurs at both sides of the InN/Si junction.It is seen that large densities of electrons accumulate at the InN/Si junction interface,which can readily recombine with holes in the Si valence band and therefore explains the quasi-ohmic behavior observed for the n-InN/i-InN/p-Si heterojunction device under dark conditions. However,the depletion creates a significant barrier to the trans-port of photogenerated holes,leading to negligible response underillumination.Fig.8.(a)I–V response of InN:Si/i-InN nanowire devices on p-Si(111) measured under dark conditions.(b)Schematic of the energy band diagram of the InN:Si/i-InN/p-Si heterojunction under thermal equilibrium.The presence of a thin(∼2to3nm)amorphous SiN x layer is alsoillustrated.Fig.9.(a)I–V characteristics of InN:Mg/i-InN/InN:Si nanowire solar cells grown on n-type Si(111)substrates under dark and illumination(1-sun at AM 1.5G)conditions.(b)Illustration of the corresponding energy band diagram of the nanowire junction under thermal equilibrium.Drastically improved device performance,illustrated in Fig.9(a),has been measured for the second solar cell de-sign grown on n-type Si substrates.Under dark conditions,the InN:Si/i-InN/InN:Mg nanowire device exhibits characteristics of a diode structure,with a significantly improved rectifying ratio(∼60)measured at–1and+1V,which is attributed to the built-in electricfield in the p-i-n nanowire homojunction.Fig.10.I–V characteristics of CdS-passivated InN:Mg/i-InN/InN:Si nanowire solar cells grown on n-type Si(111)substrates under dark and light (1-sun at AM1.5G)illumination conditions.In addition,a clear photoresponse has been consistently ob-served.Under simulated AM1.5G illumination,a promising short-circuit current density of∼12.91mA/cm2is obtained for a device area of1mm2.The measured open-circuit voltage,fill factor,and power-conversion efficiency are∼0.13V,30.2%, and0.51%,respectively.The functioning of the InN p-i-n axial homojunction solar cells on n-type Si may be explained by the energy-band diagram under thermal equilibrium.Illustrated in Fig.9(b),it may be noted that the depletion region widths of the InN/Si junction are small,due to the very high doping concen-trations in InN and Si.As a consequence,electrons can readily tunnel from InN to Si.Illumination from the top of the nanowires would result in photons with energy larger than E g(InN)to be absorbed by the nanowires.The holes and electrons produced in InN can be promptly collected by the ohmic contacts at the top of the wires and the back contact of the substrate,respectively, thereby generating the observed photocurrent.While photons with energy larger than E g(Si)could also be absorbed by the substrate when light illuminates onto the Si surface through the gap between the wires,the contribution to the photocurrent is expected to be negligible,due to the presence of a significant barrier to hole transport across the InN/Si heterointerface. The performance of the afore-described InN:Si/i-InN/ InN:Mg nanowire homojunction solar cells may be severely lim-ited by the presence of surface states,which can be addressed,to a certain extent,with the use of CdS surface passivation[46].Il-lustrated in Fig.10are the measured I–V curves under dark and illuminated conditions for the third nanowire solar cell design, wherein a thin(∼10nm)CdS passivation layer is incorporated.A reduction in the reverse leakage current and an improvement in the rectifying ratio(∼150)were measured,compared to iden-tical devices fabricated without the use of any CdS passivation. The improved diode characteristics are attributed to the effec-tive carrier confinement provided by the large bandgap CdS and the suppression of carrier leakage through the wire surface.Un-der one-sun(AM1.5G)illumination,the devices exhibit further improved performance,with a short-circuit current density of ∼14.4mA/cm2,open-circuit voltage of0.14V,fill factor of 34.0%,and energy-conversion efficiency of0.68%.It may be noted that the measured short-circuit current densities are much larger than the commonly reported values for nanowire solar cells[12],[13],[47]–[49].The open-circuit voltage,however,is relatively low,which can be improved by utilizing large bandgap InGaN nanowires and by optimizing the surface passivation and fabrication processes.The performance of the presently demonstrated InN nanowire solar cells may also be severely limited by the surface electron accumulation of n-type InN and the nonideal carrier transport across the InN/Si misfit interface,due to the presence of an amorphous SiN x layer.Improved device performance is,there-fore,expected by utilizing core/shell heterostructures and by employing a planar GaN or InN buffer layer.Additionally,the energy-conversion efficiency is practically limited by the very low wire density in this experiment.Shown in Fig.7(a),the sur-face coverage of InN nanowires is less than30%.As a result,a significant portion of the solar radiation cannot be absorbed by InN,and the benefit of light trapping associated with nanowires may be absent as well.Consequently,by optimizing the wire density and diameters,the energy-conversion efficiency can be readily increased by a factor of3,or larger.More importantly, the energy-conversion efficiency is expected to improve substan-tially for InGaN nanowire solar cells with an optimized energy bandgap.The use of coalescent growth for a planar top contact layer will also greatly facilitate the device fabrication and re-duce the series resistance[50].The growth and characterization of high-performance InGaN nanowire solar cells on Si,with the use of optimized surface passivation and device fabrication processes,are being investigated.V.C ONCLUSIONIn summary,we have investigated the MBE growth and char-acterization of high quality InN nanowires on Si(111)substrates and achieved nearly intrinsic InN nanowires as well as Si-and Mg-doped InN nanowires with excellent morphological and op-tical properties.We have further demonstrated thefirst InN solar cells,consisting of InN:Si/i-InN/InN:Mg nanowire homojunc-tions on n-type Si(111)substrates,which exhibit a promising short-circuit current density of∼14.4mA/cm2and an energy-conversion efficiency of∼0.68%under one-sun(AM1.5G) illumination.Further improvement in the device performance is being investigated by optimizing the growth and fabrication processes.The present work constitutes important progress for the realization of InGaN-based third generation solar cells.It has also mitigated some of the major barriers for the future develop-ment of InN-based nanoelectronic and nanophotonic devices.A CKNOWLEDGMENTPart of the work was performed in the McGill Nanotools Microfab Laboratory.R EFERENCES[1]J.Wu,W.Walukiewicz,K.M.Yu,W.Shan,J.W.Ager,E.E.Haller,H.Lu,W.J.Schaff,W.K.Metzger,and S.Kurtz,“Superior radiation resistance of In1−x Ga x N alloys:Full-solar-spectrum photovoltaic material system,”J.Appl.Phys.,vol.94,pp.6477–6482,Nov.15,2003.[2]O.Jani,I.Ferguson,C.Honsberg,and S.Kurtz,“Design and characteri-zation of GaN/InGaN solar cells,”Appl.Phys.Lett.,vol.91,pp.132117-1–1132117-3,Sep.24,2007.[3]H.Hamzaoui,A.S.Bouazzi,and B.Rezig,“Theoretical possibilities ofIn x Ga1−x N tandem PV structures,”Sol.Energ.Mat.Sol.Cells.,vol.87, pp.595–603,May2005.[4]X.M.Cai,S.W.Zeng,and B.P.Zhang,“Fabrication and characterizationof InGaN p-i-n homojunction solar cell,”Appl.Phys.Lett.,vol.95, pp.173504-1–173504-3,Oct.26,2009.[5]L.Hsu and W.Walukiewicz,“Modeling of InGaN/Si tandem solar cells,”J.Appl.Phys.,vol.104,pp.024507-1–024507-7,Jul.15,2008.[6]J.Wu,W.Walukiewicz,W.Shan,K.Yu,J.Ager,S.Li,E.Haller,H.Lu,and W.Schaff,“Temperature dependence of the fundamental band gap of InN,”J.Appl.Phys.,vol.94,pp.4457–4460,Oct.1,2003.[7] C.Neufeld,N.Toledo,S.Cruz,M.Iza,S.DenBaars,and U.Mishra,“Highquantum efficiency InGaN/GaN solar cells with2.95eV band gap,”Appl.Phys.Lett.,vol.93,pp.143502-1–143502-3,Oct.6,2008.[8] E.Trybus,G.Namkoong,W.Henderson,S.Burnham,W.Doolittle,M.Cheung,and A.Cartwright,“InN:A material with photovoltaic promise and challenges,”J.Cryst.Growth,vol.288,pp.218–224,Mar.1,2006.[9]X.B.Zhang,X.L.Wang,H.L.Xiao,C.B.Yang,J.X.Ran,C.M.Wang,Q.F.Hou,J.M.Li,and Z.G.Wang,“Theoretical design and performance of In x Ga1−x N two-junction solar cells,”J.Phys.D:Appl.Phys.,vol.41, p.245104,Dec.21,2008.[10] C.H.Henry,“Limiting efficiencies of ideal single and multiple energygap terrestrial solar cells,”J.Appl.Phys.,vol.51,pp.4494–4500,Aug.1980.[11]H.Neff,O.Semchinova,A.Lima,A.Fillmonov,and G.Holzhueter,“Photovoltaic properties and technological aspects of In1−x Ga x N/Si,Ge (0<x<0.6)heterojunction solar cells,”Sol.Energ.Mat.Sol.Cells., vol.90,pp.982–997,May5,2006.[12]Y.Tang,Z.Chen,H.Song,C.Lee,H.Cong,H.Cheng,W.Zhang,I.Bello,and S.Lee,“Vertically aligned p-type single-crystalline GaNnanorod arrays on n-type Si for heterojunction photovoltaic cells,”Nano Lett.,vol.8,pp.4191–4195,Dec.2008.[13]Y.J.Dong,B.Z.Tian,T.J.Kempa,and C.M.Lieber,“Coaxial groupIII-nitride nanowire photovoltaics,”Nano Lett.,vol.9,pp.2183–2187, May2009.[14]K.H.Yu and J.H.Chen,“Enhancing solar cell efficiencies through1-Dnanostructures,”Nanoscale Res.Lett.,vol.4,pp.1–10,Jan.2009. [15]Z.Cai,S.Garzon,M.Chandrashekhar,R.Webb,and G.Koley,“Synthesisand properties of high-quality InN nanowires and nanonetworks,”J.Electron.Mater.,vol.37,pp.585–592,May2008.[16] C.Chao,J.Chyi,C.Hsiao,C.Kei,S.Kuo,H.Chang,and T.Hsu,“Catalyst-free growth of indium nitride nanorods by chemical-beam epitaxy,”Appl.Phys.Lett.,vol.88,pp.233111-1–233111-3,Jun.5,2006.[17] C.Liang,L.Chen,J.Hwang,K.Chen,Y.Hung,and Y.Chen,“Selective-area growth of indium nitride nanowires on gold-patterned Si(100)sub-strates,”Appl.Phys.Lett.,vol.81,pp.22–24,Jul.1,2002.[18]T.Stoica,R.Meijers,R.Calarco,T.Richter,and H.Luth,“MBE growthoptimization of InN nanowires,”J.Cryst.Growth,vol.290,pp.241–247, Apr.15,2006.[19]J.Grandal,M.Sanchez-Garcia,E.Calleja,E.Luna,and A.Trampert,“Ac-commodation mechanism of InN nanocolumns grown on Si(111)sub-strates by molecular beam epitaxy,”Appl.Phys.Lett.,vol.91,pp.021902-1–021902-3,Jul.9,2007.[20]J.Segura-Ruiz,N.Garro,A.Cantarero,C.Denker,J.Malindretos,andA.Rizzi,“Optical studies of MBE-grown InN nanocolumns:Evidence ofsurface electron accumulation,”Phys.Rev.B,vol.79,pp.115305-1–115305-9,Mar.2009.[21]T.Stoica,R.Meijers,R.Calarco,T.Richter,E.Sutter,and H.Luth,“Pho-toluminescence and intrinsic properties of MBE-grown InN nanowires,”Nano Lett.,vol.6,pp.1541–1547,Jul.12,2006.[22]V.Darakchieva,T.Hofmann,M.Schubert,B.E.Sernelius,B.Monemar,P.O.A.Persson,F.Giuliani,E.Alves,H.Lu,and W.J.Schaff,“Free electron behavior in InN:On the role of dislocations and surface electron accumulation,”Appl.Phys.Lett.,vol.94,pp.022109-1–022109-3,Jan.12,2009.[23]M.Feneberg,J.Daubler,K.Thonke,R.Sauer,P.Schley,and R.Goldhahn,“Mahan excitons in degenerate wurtzite InN:Photolumines-cence spectroscopy and reflectivity measurements,”Phys.Rev.B,vol.77, pp.245207-1–245207-6,Jun.2008.[24] A.Janotti and C.G.Van de Walle,“Sources of unintentional conductivityin InN,”Appl.Phys.Lett.,vol.92,pp.032104-1–032104-3,Jan.21,2008.[25] C.G.Van de Walle and J.Neugebauer,“Universal alignment of hydrogenlevels in semiconductors,insulators and solutions,”Nature,vol.423, pp.626–628,Jun.5,2003.[26] E.Calleja,J.Grandal,M.Sanchez-Garcia,M.Niebelschutz,V.Cimalla,and O.Ambacher,“Evidence of electron accumulation at nonpolar sur-faces of InN nanocolumns,”Appl.Phys.Lett.,vol.90,pp.262110-1–262110-3,Jun.25,2007.[27]J.H.Song,T.Akiyama,and A.J.Freeman,“Stabilization of bulk p-typeand surface n-type carriers in Mg-doped InN{0001}films,”Phys.Rev.Lett.,vol.101,pp.186801-1–186801-4,Oct.31,2008.[28]P.A.Anderson,C.H.Swartz,D.Carder,R.J.Reeves,S.M.Durbin,S.Chandril,and T.H.Myers,“Buried p-type layers in mg-doped InN,”Appl.Phys.Lett.,vol.89,pp.184104-1–184104-3,Oct.30,2006. [29]X.Q.Wang,S.B.Che,Y.Ishitani,and A.Yoshikawa,“Growth andproperties of Mg-doped in-polar InNfilms,”Appl.Phys.Lett.,vol.90, pp.201913-1–201913-3,May14,2007.[30]R.Kudrawiec,T.Suski,J.Serafinczuk,J.Misiewicz,D.Muto,andY.Nanishi,“Photoreflectance of InN and InN:Mg layers:An evidence of Fermi level shift toward the valence band upon Mg doping in InN,”Appl.Phys.Lett.,vol.93,pp.131917-1–131917-3,Sep.29,2008.[31]R.E.Jones,K.M.Yu,S.X.Li,W.Walukiewicz,J.W.Ager,E.E.Haller,H.Lu,and W.J.Schaff,“Evidence for p-type doping of InN,”Phys.Rev.Lett.,vol.96,pp.125505-1–125505-4,Mar.31,2006.[32]I.Mahboob,T.Veal,C.McConville,H.Lu,and W.Schaff,“Intrinsicelectron accumulation at clean InN surfaces,”Phys.Rev.Lett.,vol.92, pp.036804-1–036804-4,Jan.23,2004.[33] C.L.Wu,H.M.Lee,C.T.Kuo,C.H.Chen,and S.Gwo,“Absence ofFermi-level pinning at cleaved nonpolar InN surfaces,”Phys.Rev.Lett., vol.101,pp.106803-1–106803-4,Sep.5,2008.[34] B.Beaumont,S.Haffouz,and P.Gibart,“Magnesium induced changes inthe selective growth of GaN by metalorganic vapor phase epitaxy,”Appl.Phys.Lett.,vol.72,pp.921–923,Feb.23,1998.[35] F.Furtmayr,M.Vielemeyer,M.Stutzmann,J.Arbiol,S.Estrade,F.Peiro,J.R.Morante,and M.Eickhoff,“Nucleation and growth of GaN nanorods on Si(111)surfaces by plasma-assisted molecular beam epitaxy—The influence of Si-and Mg-doping,”J.Appl.Phys.,vol.104,pp.034309-1–034309-7,Aug.1,2008.[36]Y.L.Chang,F.Li,A.Fatehi,and Z.Mi,“Molecular beam epitaxialgrowth and characterization of non-tapered InN nanowires on Si(111),”Nanotechnology,vol.20,p.345203,Aug.26,2009.[37]Y.L.Chang,F.Li,and Z.Mi,“Optimization of the structural and opticalquality of InN nanowires on Si(111)by molecular beam epitaxy,”J.Vac.Sci.Technol.B,vol.28,pp.C3B7–C3B11,May2010.[38]M.Moret,S.Ruffenach,O.Briot,and B.Gil,“The determination of thebulk residual doping in indium nitridefilms using photoluminescence,”Appl.Phys.Lett.,vol.95,pp.031910-1–031910-3,Jul.20,2009. [39]S.P.Fu,T.T.Chen,and Y.F.Chen,“Photoluminescent properties of InNepifilms,”Semicond.Sci.Technol.,vol.21,pp.244–249,Mar.2006. [40]Y.L.Chang,J.L.Wang,F.Li,and Z.Mi,“High efficiency green,yellow,and amber emission from InGaN/GaN dot-in-a-wire heterostructures on Si(111),”Appl.Phys.Lett.,vol.96,pp.013106-1–013106-3,Jan.4, 2010.[41] F.Qian,Y.Li,S.Gradecak,D.Wang,C.Barrelet,and C.Lieber,“Galliumnitride-based nanowire radial heterostructures for nanophotonics,”Nano Lett.,vol.4,pp.1975–1979,Oct.2004.[42]H.Du,I.Shih,and C.Champness,“Monocrystalline CulnSe(2)photo-voltaic cell of superior performance,”J.Vac.Sci.Technol.A,vol.22, pp.1023–1026,May/Jun.2004.[43]K.S.Ramaiah,V.S.Raja,A.K.Bhatnagar,R.D.Tomlinson,R.D.Pilkington,A.E.Hill,S.J.Chang,Y.K.Su,and F.S.Juang,“Optical, structural and electrical properties of tin doped indium oxide thinfilms prepared by spray-pyrolysis technique,”Semicond.Sci.Technol.,vol.15, pp.676–683,Jul.2000.[44]M.A.Contreras,B.Egaas,K.Ramanathan,J.Hiltner,A.Swartzlander,F.Hasoon,and R.Noufi,“Progress toward20%efficiency in Cu(In,Ca)Se-2polycrystalline thin-film solar cells,”Prog.Photovolt:Res.Appl.,vol.7, pp.311–316,Jul./Aug.1999.[45]J.S.Li,H.Y.Yu,S.M.Wong,X.C.Li,G.Zhang,P.G.Q.Lo,andD.L.Kwong,“Design guidelines of periodic Si nanowire arrays for solarcell application,”Appl.Phys.Lett.,vol.95,pp.243113-1–243113-3, Dec.14,2009.[46]O.V.Galan,rramendi,I.Riech,G.Pena,A.Iribarren,J.Aguilar-Hernandez,and G.Contreras-Puente,“Characterization of the passivation of CdS thinfilms grown by chemical bath deposition on InP,”Semicond.Sci.Technol.,vol.17,pp.1193–1197,Nov.2002.。
用正电子湮没方法鉴别InP半导体中的缺陷

第19卷第3期 半 导 体 学 报 V o l.19,N o.3 1998年3月 CH I N ESE JOU RNAL O F SE M I CONDU CTOR S M ar.,1998 用正电子湮没方法鉴别I nP半导体中的缺陷3陈志权 胡新文 王少阶(武汉大学物理系 武汉 430072)摘要 本文测量了各种InP样品中的正电子寿命谱,用正电子湮没率连续分布测量(CON2T I N分析)结合PA T F IT分析正电子寿命谱,肯定了在n型和半绝缘型InP中有In空位V I n和P空位V P,而在p型InP中只观察到In空位V I n.正电子寿命的温度关系表明所观察到的n型和半绝缘型中的V I n和V P以及p型InP中的V I n均为电中性.改进了常规的多普勒展宽谱仪.利用这一谱仪测量了n型及半绝缘型InP的多普勒展宽谱,结合正电子寿命测量结果,观察到在掺Fe的半绝缘型InP中存在V P2Fe络合物.PACC:7870B,6170B,71551 引言缺陷对半导体材料的光电特性有重要的影响,尤其随着器件尺寸的缩小以及多层系统的使用,这种影响也越来越重要.因此,鉴别和表征半导体中的缺陷特性是非常重要的,也是当今半导体科学中一项非常活跃的课题.InP作为一种In系 2 族化合物半导体,由于具有许多独特的性质,人们对它产生了很大的兴趣,广泛用于制作微电子学和光电器件.用它可制作比GaA s更好的耿氏二极管.近来,由于长波光纤通讯技术的发展,要求111~116Λm范围内的光源和接收器.而在InP衬底上生长InGaA sP双异质结激光器既能满足晶格匹配,又能满足波长范围的要求.另外,从禁带宽度的角度来看,InP也是很好的太阳能电池材料.尽管InP有较为广阔的应用前景,但其制备比较困难,因此目前对它的研究明显少于对GaA s的研究.正电子是电子的反粒子,它对空位型缺陷特别敏感,能够被空位所捕获,在缺陷处的寿命明显大于体态湮没寿命,且正电子谱学有很强的理论支持,实验结果可与理论进行比较, 3国家自然科学基金资助课题陈志权 男,1969年出生,博士,现从事正电子湮没谱学研究工作1997202201收到,1997206206定稿因此成为研究半导体材料缺陷特性的有力工具[1].本文中我们选择了一系列InP样品,用正电子湮没寿命谱和多普勒展宽技术进行了研究.我们的目的在于探讨:1)在InP样品中究竟存在哪些原生缺陷?2)在不同掺杂及掺杂浓度的样品中这些缺陷是否有变化?3)能否用正电子湮没方法鉴别InP中的空位2杂质复合体?2 实验方法所有InP样品均为液态密封直拉法(L EC)生长所得单晶样品,切成10×10×015mm3的片状,用于正电子湮没测量.正电子寿命谱仪采用快2快符合谱仪,每谱计数2×106用于图1 改进的多普勒展宽装置示意图PA T F IT[2]程序分析,计数107则用于CON T I N[3,4]程序分析.从标准样品Si 的寿命测量中可得出源成分为10%的165p s和013%的1000p s.改进的多普勒展宽装置如图1所示,采用两台Χ光子探测器,一台为高纯锗探测器(H P2Ge),另一台为N a I (T l)探测器,两探头输出信号经放大后送入单道分析器(SCA),进行能量选择并送入符合器.在我们的实验中,只选择01511M eV的湮没辐射Χ光子.经此改装后的装置只收集正电子湮没辐图2 利用改进的多普勒展宽谱仪所测谱与用常规谱仪对比射Χ光子,这样可完全去掉环境辐射的影响[5],使本底大大降低.图2示出了我们利用这一改进谱仪所测的多普勒展宽谱.由图可以看出,无符合的谱仪其峰对本底之比为150∶1,采用符合后则升为2500∶1,提高了一个数量级,因此增加了谱线型参数的精度.3 结果讨论及分析3.1 缺陷鉴别我们首先测量了四种InP样品,一种n型,一种p型和两种S I型,CON2T I N分析数值结果列于表1中,所得湮没率分布示于图3中,由于两种S I型样品分布基本相同,图中只给出三种导电类型不同的样品的结果.681 半 导 体 学 报 19卷表1 lnP 中正电子湮没率连续分布结果掺杂导电类型载流子浓度或电阻率〈Κ〉 ns -1〈Κ-1〉 ns A RD 范围 ns -1FW HM ns -1S n 1.4×1019c m -34.06260.24783.52~4.560.3019Fe S I 2×1078・c m4.08630.24653.52~4.560.3090Fe S I 7×1068・c m 4.08650.24653.52~4.560.3065Znp3.9×1018c m-34.14630.24293.78~4.560.2338 〈Κ〉为平均湮没率,〈Κ-1〉为平均寿命,FW HM 为半高宽. 从图3和表1的结果我们可以观察到:1)对于n 型和S I 型样品,正电子湮没率分布完全相同,不受掺杂元素或载流子浓度的影响,平均寿命也相同,即为247p s ,并且分布宽度FW HM 都约为013050n s -1.2)对p 型图3 InP 样品中正电子湮没率分布(A RD )Κ为湮没率,ΚΑ(Κ)为湮没率的几率.InP ,湮没率分布则明显发生了变化.首先,峰中心位置朝较高值方向移动,平均寿命下降为243p s ,其次,峰的分布宽度也变得窄多了,其FW HM 为012338n s -1.这反映了在这些样品中,缺陷特性也不相同.我们还系统测量了一批不同样品的正电子寿命谱,并用PA T F IT 程序进行分析.结果见表2.对于所有的寿命谱,均可分解出两个分量.根据两态捕获模型,计算出体寿命Σb =240p s .由于半导体中缺陷态寿命比体寿命仅高出10%~20%,因此分解正电子寿命谱比较困难,其结果往往不可靠.根据H au to jarvi 的建议,平均寿命是研究缺陷特性变化的很好的参数[1],因此我们只给出了平均寿命的结果.由表中可以看出,所有的样品中平均寿命均大于体寿命,因此证实了存在空位型缺陷.在n 型和S I 型InP 中,平均寿命值为247p s 左右,而在p 型InP 中,平均寿命下降为243p s 左右,与CON T I N 分析结果相同.在两种样品中,平均寿命均不受载流浓度的影响.表2 I nP 样品特性及PATF IT 分析的正电子寿命测量结果样品号掺杂导电类型载流子浓度或电阻率平均寿命Σmp s 1N one n 2×1016c m -3247.32S n 8×1017c m-3247.23S n 4.87×1018c m -3246.84S n 1.4×1019c m-3247.55S n (4~5)×1019c m -3247.16Zn p 2.68×1018c m -3242.87Zn p 3.9×1018c m -3243.18Fe S I 6×1078・c m 247.49Fe S I 6×1078・c m246.910Fe S I 4×1078・c m 247.111Fe S I 2×1078・c m248.112FeS I7×1068・c m246.6由于所有样品均是在同一条件下生长的,只是掺杂引起费米能级位置不同,因此导致缺7813期 陈志权等: 用正电子湮没方法鉴别InP 半导体中的缺陷 陷特性(如电荷态)的变化.在InP中正电子可能有以下湮没态,即1)非局域的自由正电子态,即布洛赫态;2)在P空位处的捕获态;3)在In空位处的捕获态.图4所示的湮没率分布代表了这些湮态的组合.B retagnon等人[6]的结果表明在p型InP中存在In空位捕获正电子,因此我们可认为p型中e+湮没态为体态和In空位捕获态.P空位可能带正电荷,不捕获正电子.在n型和S I型InP中相对于p型样品显然增加了正电子态,这一湮没态则为P 空位捕获态,即In空位和P空位均捕获正电子.根据理论计算的缺陷电离能级结果[7],在n型和S I型中In空位和P空位均可带中性或负电荷态,即有可能捕获正电子,而在p型InP中V P有可能带正电荷,不能捕获正电子.这表明我们的正电子结果与理论计算是相符的.我们选择了一种n型(未掺杂)InP,测量了正电子寿命谱的温度关系.平均寿命Σm随温图4 n2InP中正电子平均寿命随温度的变化度的变化示于图4中.结果发现在所测温度范围内平均寿命保持不变.根据捕获模型,k= (Σm-Σb) [Σb(Σd-Σm)],Σd、Σb是固定的,因此从Σm不随温度变化,即可得出捕获率k也不随温度变化.B retagnon等人[6]也测量了所有InP 样品正电子寿命的温度关系,其温度范围从30K到300K,且同样发现寿命不受温度的影响,根据Pu ska的理论计算[8],中性空位的正电子捕获率与温度无关,因此结合这些结果,我们认为所探测到的In空位和P空位均为电中性.3.2 缺陷-杂质络合物研究利用改进的多普勒展宽谱仪测量了五种样品的多普勒展宽谱,结果列于表3中.可以明显看出,在同一导电类型的样品中,S参数和W参数均基本相同,但不同导电类型样品中的S参数或W参数有明显差别.在n型InP中,S参数为015203,而在S I型InP中,却降低至015184.这一变化量为误差∆S(010005)的四倍,因此是可信的.对于W参数,在n型中为010325,而在S I型InP中为010330,变化量为误差∆W(9×10-5)的五倍.考虑到这些样品中唯一的差别就是掺杂元素的不同,因此引起了S参数和W参数变化的可能因素是所掺的Fe杂质原子,改变了电子动量密度分布.表3 I nP中的多普勒展宽线型参数测量结果序号掺杂导电类型电阻率 (8・c m)载流子浓度 c m-3Σm p s W参数S参数1none n2×1016247.30.03260.5201 2S n3.2×1018247.30.03240.5205 3Fe S I~6×107247.40.03270.5187 4Fe S I~6×107246.90.03310.5185 5Fe S I~2×107248.10.03320.5180S参数的误差为5×10-4,W参数的误差为9×10-5 根据正电子捕获模型,S参数和W参数可以表达成如下形式:S=(1-f)S b+f S d W=(1-f)W b+f W d(1) 881 半 导 体 学 报 19卷其中 S b (W b )为体态S (W )参数;S d (W d )为缺陷态的S (W )参数;f 为正电子被空位捕获的比例,且f 为:f =k (Κb +k )(2)式中 k 为正电子捕获率;Κb 为体态湮没率.由寿命结果可知Σm 相同,因此推出比例f 也相同.由于Fe 原子掺杂浓度在1016~1017c m -3之间,而In 原子及P 原子的浓度为1022c m -3,Fe 原子的浓度远远小于P 原子或In 原子的浓度,很显然,它对S b (W b )的影响将是可以忽略的.因此我们认为Fe 原子位于空位的附近,对S d (W d )产生影响,改变S 参数和W 参数.正电子在空位处的波函数有一部分会扩展至周围原子间隙,从而与周围原子发生交迭[9].如果空位附近有杂质原子,它将改变湮没辐射线型参数,其改变程度取决于杂质原子的外层电子组态.Fe 原子掺入 2 族化合物半导体后,通常占据 族元素原子位置.此时Fe 原子可表现出三种不同的价态,其最外层电子组态均为3d ,电子具有较高动量,而InP 中P 原子外层电子组态为3s 3p ,In 原子的则为5s 5p ,其电子动量较低.因此,若正电子在空位处与Fe 原子的外层电子发生湮没,其辐射线型将会变宽,而在n 型InP 中,多普勒线型均比较窄,我们观察到对n 型InP 样品,S 参数确实要大些(015203),W 参数要小些(010325),这说明我们的分析是完全正确的.实际上,早期的光致发光(PL )谱已经显示了与Fe 有关的缺陷复合体存在的证据[10~12].并认为可能是Fe In 2V P 复合体.我们利用多普勒展宽技术给出了在掺Fe 的InP 中存在V P 2Fe 络合物的直接证据.4 结论(1)在n 型和S I 型InP 中存在In 空位和P 空位,而在p 型InP 中只观察到In 空位,且这些空位的电荷态均为中性.(2)利用改进的多普勒展宽谱仪,在掺Fe 的S I 2InP 中发现了V P 2Fe 络合物.参考文献[1] P .H auto ja βrvi ,M ater .Sci .Fo rum ,1995,175~178:47.[2] PA T F IT package ,R is ΥN ati onal L abo rato ry ,Ro sk ide ,D enm ark ,1989.[3] R .B .Grego ry and Y .Zhu ,N ucl .Instrum .M ethods Phys .R es .,A ,1990,290:172.[4] Z .T ang and S .J .W ang ,N ucl .Instr .M eth .,A ,1995,355:548.[5] K .G .L ynn and A .N .Go land ,So lid State Comm un .,1976,18:1549.[6] T .B retagnon ,S .D annefaer and D .Kerr ,J .A pp l .Phys .,1993,73:4697.[7] M .A latalo ,R .M .N iem inen ,M .J .Puska et a l .,Phys .R ev .,B ,1993,47:6381.[8] M .J .Puska ,C .Co rbel and R .M .N iem inen ,Phys .R ev .,B ,1990,41:9980.[9] M .J .Puska ,S .M ak innen ,M .M anninen et a l .,Phys .R ev .,B ,1989,39:7666.[10] Yu .P .W on ,in Sem i 2Insulating 2 m aterials (Sh iva ,O rp ington ),eds .G .J .R eesleds ,1980,167.[11] Y .Yam azoe ,Y ,Sasai ,T .N ish ino et al .,Jpn .J .A pp l .Phys .,1981,20:347.[12] K .Kuriyam a ,K .Tom izaw a and M .Kash i w akura ,J .A pp l.Phys .,1994,76:3552.9813期 陈志权等: 用正电子湮没方法鉴别InP 半导体中的缺陷 091 半 导 体 学 报 19卷D iscr i m i na tion of D efects i n I nP Sem iconductorsby Positron Ann ih ila tionChen Zh iquan,H u X inw en and W ang Shao jie(D ep art m en t of P hy sics,W uhan U niversity,W uhan 430072)R eceived1February1997,revised m anuscri p t received6June1997Abstract Po sitron lifeti m e sp ectra have been m easu red fo r vari ou s InP sam p les,and ana2 lyzed by CON T I N p rogram as w ell as PA T F IT p rogram to ob tain the con tinuou s po sitron ann ih ilati on distribu ti on.T he resu lts assu re that In vacancy and P vacancy ex ist in n2and Sem i2in su lating InP,bu t on ly In vacancy is ob served in p typ e InP.T he tem p eratu re be2 havi o r of po sitron lifeti m e show s that the V In,V P in n2and S I2InP and V I n in p2InP are neu2 trally charged.W e have revised the conven ti onal Dopp ler B roaden ing sp ectrom eter and m easu red the DB sp ectra fo r n2and S I2typ e InP sam p b ined w ith the resu lt of po sitron lifeti m e,w e have ob served the V P2Fe com p lexes in Fe dop ed S I2InP.PACC:7870B,6170B,7155。
磁性壳聚糖纳米介导eNOS基因转染受损平滑肌细胞初探
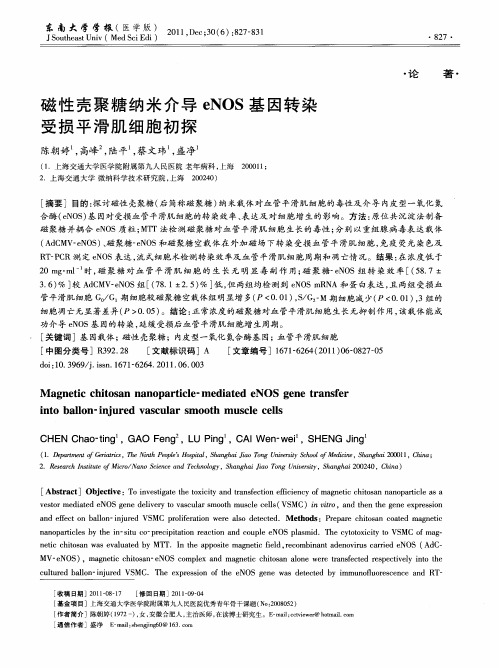
・
8 27 ・
磁 性壳聚糖纳米介导 e NOS基 因转 染 受 损 平 滑肌 细胞 初 探
陈朝婷 高峰 陆平 蔡文玮 盛净 , , , ,
( .上海交通大学医学 院附属第九人 民医院 老年病科 , 1 上海
2 .上 海 交 通 大 学 微 纳 科 学 技 术 研 究 院 , 海 上 204 ) 02 0
a de et n bl n i ue S rleai eea od t t .M eh d :Peaec i sncae an t n f c o a o —n rdV MC poi rt n w r l ee e f l j f o s cd to s rp r ht a ot m gei o d c
(.Dp r etfGr tc, h i hP ols o i l S ag a J oTn n e i colfM d ie S nhi 00 1 C ia 1 eat n o ears Te n e e s t , hnh i i ogU i rt Sho o ewn , h ga 0 1 , hn ; m i i N t p 'H p a a v sy a 2 2 eerhIstt o coN n c nea dTcn l y S ag a ioTn n e i , h nh i 0 20 hn ) .Rsac tuefMi / a oSi c n ehoo , h n h i a og U i rt S a g a 2 0 4 ,C i ni r e g J v sy a
[ s at Ab t c]0bet e T vsg t tet ii n as c o fc nyo g e ce i snnn p rc s r jc v : oi et a xc ya dt nf t ne i c f nt ht a ao at l a i n i eh o t r ei i e ma i o ie a
超交联有机聚合物的合成及其吸附性能应用进展
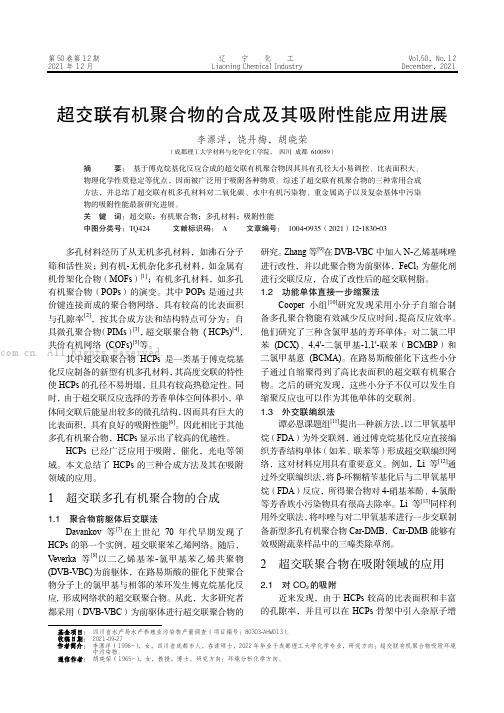
第50卷第12期 辽 宁 化 工 Vol.50,No. 12 2021年12月 Liaoning Chemical Industry December,2021基金项目: 四川省水产局水产养殖业污染物产量调查(项目编号:80303-AHW013)。
收稿日期: 2021-09-27 作者简介: 李漂洋(1996-),女,四川省成都市人,在读硕士,2022年毕业于成都理工大学化学专业,研究方向:超交联有机聚合物吸附环境中污染物。
通信作者: 胡晓荣(1965-),女,教授,博士,研究方向:环境分析化学方向。
超交联有机聚合物的合成及其吸附性能应用进展李漂洋,饶丹梅,胡晓荣(成都理工大学材料与化学化工学院, 四川 成都 610059)摘 要: 基于傅克烷基化反应合成的超交联有机聚合物因其具有孔径大小易调控、比表面积大、物理化学性质稳定等优点,因而被广泛用于吸附各种物质。
综述了超交联有机聚合物的三种常用合成方法,并总结了超交联有机多孔材料对二氧化碳、水中有机污染物、重金属离子以及复杂基体中污染物的吸附性能最新研究进展。
关 键 词:超交联;有机聚合物;多孔材料;吸附性能中图分类号:TQ424 文献标识码: A 文章编号: 1004-0935(2021)12-1830-03多孔材料经历了从无机多孔材料,如沸石分子筛和活性炭;到有机-无机杂化多孔材料,如金属有机骨架化合物(MOFs )[1];有机多孔材料,如多孔有机聚合物(POPs )的演变。
其中POPs 是通过共价键连接而成的聚合物网络,具有较高的比表面积与孔隙率[2],按其合成方法和结构特点可分为:自具微孔聚合物(PIMs )[3],超交联聚合物 ( HCPs)[4],共价有机网络 (COFs)[5]等。
其中超交联聚合物HCPs 是一类基于傅克烷基化反应制备的新型有机多孔材料,其高度交联的特性使HCPs 的孔径不易坍塌,且具有较高热稳定性。
同时,由于超交联反应选择的芳香单体空间体积小,单体间交联后能显出较多的微孔结构,因而具有巨大的比表面积,具有良好的吸附性能[6]。
《氮化物半导体量子点中的束缚极化子、激子及应变效应》范文
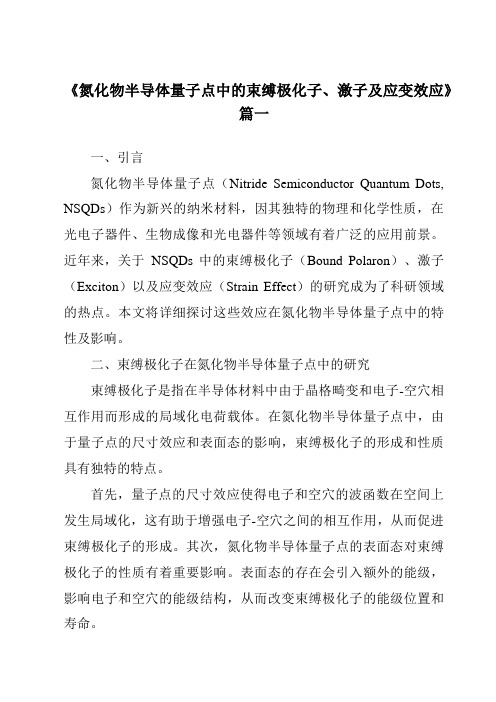
《氮化物半导体量子点中的束缚极化子、激子及应变效应》篇一一、引言氮化物半导体量子点(Nitride Semiconductor Quantum Dots, NSQDs)作为新兴的纳米材料,因其独特的物理和化学性质,在光电子器件、生物成像和光电器件等领域有着广泛的应用前景。
近年来,关于NSQDs中的束缚极化子(Bound Polaron)、激子(Exciton)以及应变效应(Strain Effect)的研究成为了科研领域的热点。
本文将详细探讨这些效应在氮化物半导体量子点中的特性及影响。
二、束缚极化子在氮化物半导体量子点中的研究束缚极化子是指在半导体材料中由于晶格畸变和电子-空穴相互作用而形成的局域化电荷载体。
在氮化物半导体量子点中,由于量子点的尺寸效应和表面态的影响,束缚极化子的形成和性质具有独特的特点。
首先,量子点的尺寸效应使得电子和空穴的波函数在空间上发生局域化,这有助于增强电子-空穴之间的相互作用,从而促进束缚极化子的形成。
其次,氮化物半导体量子点的表面态对束缚极化子的性质有着重要影响。
表面态的存在会引入额外的能级,影响电子和空穴的能级结构,从而改变束缚极化子的能级位置和寿命。
三、激子在氮化物半导体量子点中的研究激子是指在半导体材料中由光激发或电激发产生的电子-空穴对。
在氮化物半导体量子点中,激子的性质与传统的体材料有所不同,主要表现在激子的能级结构和动力学行为上。
由于量子点的尺寸效应,激子的能级结构在空间上发生局域化,这有助于增强激子的稳定性。
此外,氮化物半导体量子点的表面态也会影响激子的动力学行为。
表面态可以作为电子和空穴的陷阱,影响激子的产生、复合以及与其他载流子的相互作用。
四、应变效应在氮化物半导体量子点中的应用应变效应是指在外界应力作用下,半导体材料的晶格发生形变,从而影响材料的电子结构和物理性质。
在氮化物半导体量子点中,应变效应对材料的电子结构和光学性质有着显著的影响。
通过施加应力,可以调控氮化物半导体量子点的能带结构,从而改变其光学性质。
《噪声与振动控制技术手册》已出版发行

modeling
of
membrane-type
acoustic
metamaterials: plate model[J]. Journal of the Acoustical
Society of America, 2014, 136(3): 2926.
[10] LANGFELDT F, GLEINE W, ESTORFF O V. Analytical
243-247.
[12] MA G, YANG M, YANG Z, et al. Low-frequency narrowband acoustic filter with large orifice[J]. Applied Physics
Letters, 2013, 103(1): 851-R.
model for low-frequency transmission loss calculation of
membranes loaded with arbitrarily shaped masses[J].
Journal of Sound & Vibration, 2015, 349: 315-329.
????????????????????????????????????????????????????????????????????????????????????????????????????????????????????????????????????????????????????????????????????????????????????????????书讯噪声与振动控制技术手册已出版发行由中船第九设计研究院工程有限公司牵头联合清华大学北京市劳动保护科学研究所组织编写的噪声与振动控制技术手册主编吕玉恒副主编燕翔魏志勇邵斌孙家麒冯苗锋已由化学工业出版社于2019年9月出版发行
am晶格转变和沉淀析出

AM晶格转变和沉淀析出1. 介绍AM晶格转变和沉淀析出是材料科学领域中的重要研究课题。
在晶体学中,晶格转变是指晶体结构发生变化,而沉淀析出是指溶液中的溶质在适当条件下形成固态颗粒。
这两个过程在材料制备和性能改善中起着关键作用。
2. AM晶格转变AM晶格转变是指晶体中的原子或离子的排列方式发生变化,从而导致晶体结构的改变。
这种转变可以通过温度、压力、化学条件等外界因素来引发。
晶格转变的研究对于理解材料的性质和改善其性能具有重要意义。
晶格转变可以导致晶体的相变。
相变是指物质从一种相态转变为另一种相态的过程。
常见的相变包括固态相变、液态相变和气态相变。
晶格转变可以引发固态相变,从而改变晶体的物理和化学性质。
晶格转变的研究方法主要包括X射线衍射、电子显微镜和拉曼光谱等。
这些方法可以用来分析晶体结构的变化和晶格参数的变化。
通过研究晶格转变的机制和规律,可以揭示材料的性质和改善材料的性能。
3. 沉淀析出沉淀析出是指溶液中的溶质在适当条件下形成固态颗粒的过程。
溶质可以是固体、液体或气体。
沉淀析出的过程包括溶解、扩散和沉淀三个阶段。
溶解是指溶质在溶剂中分子或离子的解离和溶解过程。
扩散是指溶质分子或离子在溶液中的扩散和迁移过程。
沉淀是指溶质在溶液中逐渐聚集形成固态颗粒的过程。
沉淀析出的过程受到溶液浓度、温度、pH值、离子强度和搅拌速度等因素的影响。
这些因素可以通过调控实验条件来控制沉淀析出的过程和产物的性质。
沉淀析出在材料制备和性能改善中具有重要应用。
通过控制沉淀析出的条件,可以制备出具有特定形状、尺寸和组成的纳米颗粒。
这些纳米颗粒在催化、传感、能源存储等领域具有广泛的应用前景。
4. 应用AM晶格转变和沉淀析出在材料科学中有着广泛的应用。
以下列举几个典型的应用案例:4.1 材料制备通过控制晶格转变和沉淀析出的条件,可以制备出具有特定结构和性能的材料。
例如,通过调控晶格转变的温度和压力,可以制备出具有高温超导性能的材料。
一种增强N型半导体稳定性的方法[发明专利]
![一种增强N型半导体稳定性的方法[发明专利]](https://img.taocdn.com/s3/m/54053088d0f34693daef5ef7ba0d4a7303766c5c.png)
专利名称:一种增强N型半导体稳定性的方法专利类型:发明专利
发明人:李立强,苑力倩,黄忆男,胡文平
申请号:CN202210013229.3
申请日:20220107
公开号:CN114361338A
公开日:
20220415
专利内容由知识产权出版社提供
摘要:本发明公开了抗坏血酸在增强N型半导体稳定性中的应用及一种增强N型半导体稳定性的方法,属于半导体材料技术领域。
所述方法为在N型半导体器件表面构筑抗坏血酸薄膜,所述抗坏血酸薄膜的制备方法为:将抗坏血酸溶液通过悬涂法、提拉法或滴注法均匀涂布在所述N型半导体器件表面,自然固化或者真空退火固化。
所述抗坏血酸溶液中还加入聚氨酯溶液。
该方法采用氧消除的策略,抗坏血酸可以清除已加入N型半导体中的氧,消除禁带中的相关陷阱状态,防止N型半导体的进一步降解。
利用本发明的方法所制备的N型半导体器件的迁移率等电学性能提升,操作稳定性和长时间存储稳定性均得到提高。
申请人:天津大学
地址:300072 天津市南开区学府街道卫津路92号天津大学卫津路校区
国籍:CN
代理机构:北京盛询知识产权代理有限公司
代理人:李艳芬
更多信息请下载全文后查看。
胰岛素固体脂质纳米粒的制备及其包封率的测定
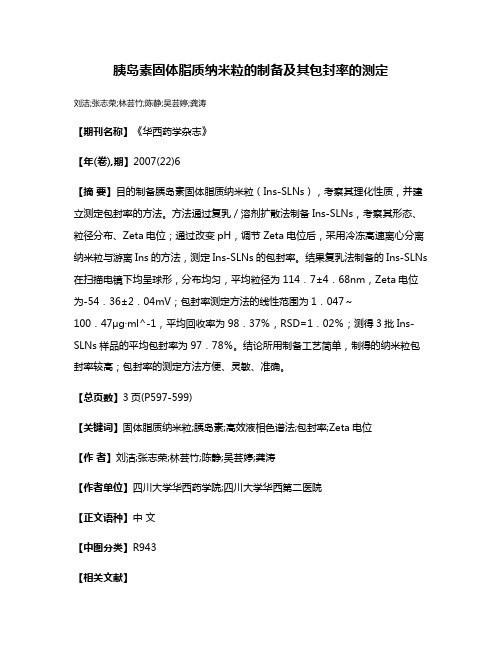
胰岛素固体脂质纳米粒的制备及其包封率的测定刘洁;张志荣;林芸竹;陈静;吴芸婷;龚涛【期刊名称】《华西药学杂志》【年(卷),期】2007(22)6【摘要】目的制备胰岛素固体脂质纳米粒(Ins-SLNs),考察其理化性质,并建立测定包封率的方法。
方法通过复乳/溶剂扩散法制备Ins-SLNs,考察其形态、粒径分布、Zeta电位;通过改变pH,调节Zeta电位后,采用冷冻高速离心分离纳米粒与游离Ins的方法,测定Ins-SLNs的包封率。
结果复乳法制备的Ins-SLNs 在扫描电镜下均呈球形,分布均匀,平均粒径为114.7±4.68nm,Zeta电位为-54.36±2.04mV;包封率测定方法的线性范围为1.047~100.47μg·ml^-1,平均回收率为98.37%,RSD=1.02%;测得3批Ins-SLNs样品的平均包封率为97.78%。
结论所用制备工艺简单,制得的纳米粒包封率较高;包封率的测定方法方便、灵敏、准确。
【总页数】3页(P597-599)【关键词】固体脂质纳米粒;胰岛素;高效液相色谱法;包封率;Zeta电位【作者】刘洁;张志荣;林芸竹;陈静;吴芸婷;龚涛【作者单位】四川大学华西药学院;四川大学华西第二医院【正文语种】中文【中图分类】R943【相关文献】1.离心法和透析法测定蟾皮提取物固体脂质纳米粒包封率的比较 [J], 蔡晓瑶;张莉;任翔;张磊2.微柱离心-HPLC法测定载左旋多巴聚乙二醇化固体脂质纳米粒的包封率 [J], 魏曼;张宇佳;陈谕园;张楠;郑稳生3.扎那米韦固体脂质纳米粒的制备及其包封率测定 [J], 陈林;刘妍;姜绍波;曹青日;崔京浩4.HPLC法测定葛根总黄酮固体脂质纳米粒的载药量及包封率 [J], 熊月路;崔蕴慧;曾天骏;巫嘉华;李冬玲;王治平;王一飞5.HPLC法测定天山雪莲提取物固体脂质纳米粒载药量与包封率 [J], 刘桂花; 刘宣麟; 帕依曼·亥米提; 何承辉因版权原因,仅展示原文概要,查看原文内容请购买。
氧化型低密度脂蛋白诱导内皮细胞表达基质细胞衍生因子1致CXCR4^+骨髓间充质干细胞迁移
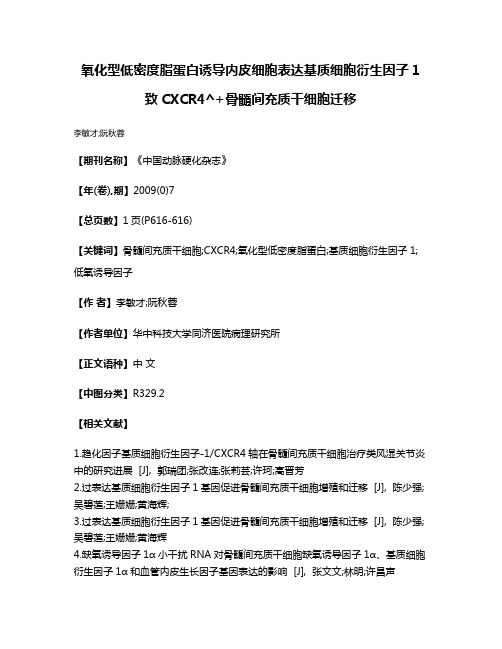
氧化型低密度脂蛋白诱导内皮细胞表达基质细胞衍生因子1致CXCR4^+骨髓间充质干细胞迁移
李敏才;阮秋蓉
【期刊名称】《中国动脉硬化杂志》
【年(卷),期】2009(0)7
【总页数】1页(P616-616)
【关键词】骨髓间充质干细胞;CXCR4;氧化型低密度脂蛋白;基质细胞衍生因子1;低氧诱导因子
【作者】李敏才;阮秋蓉
【作者单位】华中科技大学同济医院病理研究所
【正文语种】中文
【中图分类】R329.2
【相关文献】
1.趋化因子基质细胞衍生因子-1/CXCR4轴在骨髓间充质干细胞治疗类风湿关节炎中的研究进展 [J], 郭瑞团;张改连;张莉芸;许珂;高晋芳
2.过表达基质细胞衍生因子1基因促进骨髓间充质干细胞增殖和迁移 [J], 陈少强;吴碧莲;王姗姗;黄海辉;
3.过表达基质细胞衍生因子1基因促进骨髓间充质干细胞增殖和迁移 [J], 陈少强;吴碧莲;王姗姗;黄海辉
4.缺氧诱导因子1α小干扰RNA对骨髓间充质干细胞缺氧诱导因子1α、基质细胞衍生因子1α和血管内皮生长因子基因表达的影响 [J], 张文文;林明;许昌声
5.基质细胞衍生因子1及其受体CXCR4对人骨髓间充质干细胞向脑缺血损伤区迁移的影响 [J], 朱洁;周竹娟;龚自力;郑健
因版权原因,仅展示原文概要,查看原文内容请购买。
对苯二酚镧(Ⅲ)配合物的合成及光谱分析
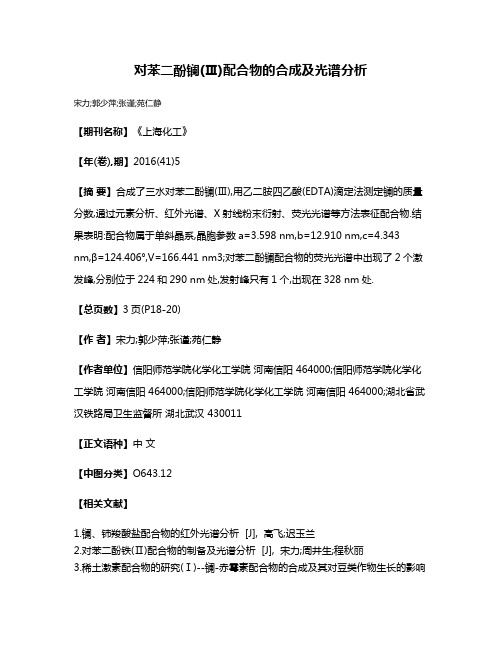
对苯二酚镧(Ⅲ)配合物的合成及光谱分析
宋力;郭少萍;张谨;苑仁静
【期刊名称】《上海化工》
【年(卷),期】2016(41)5
【摘要】合成了三水对苯二酚镧(Ⅲ),用乙二胺四乙酸(EDTA)滴定法测定镧的质量分数,通过元素分析、红外光谱、X射线粉末衍射、荧光光谱等方法表征配合物.结果表明:配合物属于单斜晶系,晶胞参数a=3.598 nm,b=12.910 nm,c=4.343 nm,β=124.406°,V=166.441 nm3;对苯二酚镧配合物的荧光光谱中出现了2个激发峰,分别位于224和290 nm处,发射峰只有1个,出现在328 nm处.
【总页数】3页(P18-20)
【作者】宋力;郭少萍;张谨;苑仁静
【作者单位】信阳师范学院化学化工学院河南信阳 464000;信阳师范学院化学化工学院河南信阳 464000;信阳师范学院化学化工学院河南信阳 464000;湖北省武汉铁路局卫生监督所湖北武汉 430011
【正文语种】中文
【中图分类】O643.12
【相关文献】
1.镧、铈羧酸盐配合物的红外光谱分析 [J], 高飞;迟玉兰
2.对苯二酚铁(Ⅱ)配合物的制备及光谱分析 [J], 宋力;周井生;程秋丽
3.稀土激素配合物的研究(Ⅰ)--镧-赤霉素配合物的合成及其对豆类作物生长的影响
[J], 滕莉丽;罗国添;练萍;郭国端
4.三个喹啉氧基乙酰胺镧系(Eu、Gd、Er)配合物的合成、结构及Eu配合物的荧光性质 [J], 毛盼东;陈亮;吴伟娜;贾磊;王元
5.镧系金属有机配合物的研究 XL.双(2-甲氧乙基环戊二烯基)钇和镱的硼氢配合物的合成及晶体结构 [J], 邓道利;郑晓帆;钱长涛;金松春;林永华
因版权原因,仅展示原文概要,查看原文内容请购买。
- 1、下载文档前请自行甄别文档内容的完整性,平台不提供额外的编辑、内容补充、找答案等附加服务。
- 2、"仅部分预览"的文档,不可在线预览部分如存在完整性等问题,可反馈申请退款(可完整预览的文档不适用该条件!)。
- 3、如文档侵犯您的权益,请联系客服反馈,我们会尽快为您处理(人工客服工作时间:9:00-18:30)。
P hilosophical M agazine ,1September 2003V ol.83,N o.25,2941–2953Intrinsic nanoscale phase separation of bulk As 2S 3glassD.G.Georgiev,P.Boolchand yDepartment of Electrical,Computer Engineering and Computer Science,University of Cincinnati,Cincinnati,Ohio 45221-0030,USAand K.A.JacksonDepartment of Physics,Central Michigan University,Mount Pleasant,Michigan 48858,USA[Received 27April 2003and accepted 20May 2003]A bstractRaman scattering on bulk As x S 1Àx glasses shows that vibrational modes ofAs 4S 4monomer first appear near x ¼0.38,and their concentration increases precipitously with increasing x ,suggesting that the stoichiometric glass (x ¼0.40)is intrinsically phase separated into small As-rich (As 4S 4)and large S-rich clusters.Support for the Raman-active vibrational modes of the orpiment-like and realgar-like nanophases is provided by ab -initio density functional theory calculations on appropriate clusters.Nanoscale phase separation provides a basis for understanding the global maximum in the glass transition temperature T g near x ¼0.40,and the departure from Arrhenius temperature activation of As 2S 3melt viscosities.}1.IntroductionOrpiment (As 2S 3)crystallizes in a layer-like structure with each layer consisting of 12-membered As 2(S 1/2)3rings (Morimoto 1954)(see also Renninger et al .(1973)),forming elements of medium-range structure.Each ring consists of six pyramidally coordinated As(S 1/2)3units with bridging S atoms.The intralayer bonding interac-tions are covalent,while the interlayer interactions are van der Waals in character and weaker.The vibrational density of states in orpiment has been examined by Raman scattering (Zallen and Slade 1974,Kawamura et al .1983).Interlayer optic modes exhibit pressure shifts (Zallen 1974)at least an order of magnitude larger (Gru neisen mode)than intralayer modes,confirming the quasi-two-dimensional molecular structure in vibrational spectroscopy.In analogy to orpiment the molecular structure of stoichiometric As 2S 3glass is widely regarded to consist of a network of S-bridging pyramidal As(S 1/2)3units (Myers and Felty 1967).Several studies have emphasized that the similarity of structure goes beyond the local order to the medium-range order;that is,the glass network also possesses a layer-like structure (Tanaka et al .1985,Tanaka 1989,Shimakawa et al .1998,Taylor et al .1998)although that view is not sharedPhilosophical Magazine ISSN 1478–6435print/ISSN 1478–6443online #2003Taylor &Francis Ltd/journalsDOI:10.1080/1478643031000151196y Author for correspondence.Email:pboolcha@.2942 D.G.Georgiev et al.universally(Yang et al.1987).Evidence for presence of a small butfinite(less than 2%)concentration of like-atom bonds(As–As and S–S)in the stoichiometric glass has emerged from Raman scattering(Frumar et al.1997,Wagner et al. 1998)and129I Mo ssbauer spectroscopy(Zitkovsky1989).An issue of central importance concerns the distribution of these like-atom bonds in the glass structure.A common view is to regard such bonds as‘defects’in a fully polymerized network.The like-atom bonds then form at random in a nearly chemically ordered but continuous random network.An alternative view(Phillips et al.1980)is to regard the As–As bonds and S–S bonds as forming part of two distinct molecular clusters which bond to each other by weaker(van der Waals) forces.In the latter model,internal surfaces become an intrinsic feature of glass structure and the network is viewed as partially polymerized,that is phase separated on a molecular scale or nanoscale.Nanoscale phase separation effects are of general interest in glass science (Boolchand et al.2002).Such structural effects usually produce pronounced changes in glass physical properties including a lowering of the glass transition temperature,a lowering of the optical bandgap and an increase in molar volumes as network global connectivity diminishes.These pronounced changes in physical properties can mask the more subtle elastic effects related to rigidity transitions as discussed recently (Boolchand et al.2002).In general,it appears that chalcogen-rich glasses are usually fully polymerized,but chalcogen-deficient glasses tend to demix into characteristic nanophases although exceptions do occur.Stoichiometric glasses(GeS2,GeSe2, As2Se3and As2S3)that are at the boundary between chalcogen-rich and chalcogen-deficient glasses have thus attracted much attention.The evidence(Bresser et al. 1981)(see also Boolchand(2000a)and Boolchand and Bresser(2000))suggests that the stoichiometric glasses of GeSe2and GeS2are composed of an anionic-rich majority phase and a cation-rich minority phase.In this work,we present new Raman scattering and temperature-modulated differential scanning calorimetry(DSC)results on binary As x S1Àx glasses focusing near the stoichiometric composition with x¼2/5.The present results in conjunction with previous129I Mo ssbauer effect results(Zitkovsky1989)show that the stoichiometric As2S3glass is nanoscale phase separated into small As4S4molecules and compensating large S-rich clusters(Phillips et al.1980).Thefinding is consistent with the global maximum of T g near the stoichiometric composition,and the non-Arrhenius temperature activation of As2S3liquid viscosities(Vinogradova et al.1967)(see also Angell(1988,1991)).}2.Experimental results99.999%As2S3and elemental S and As lumps from Cerac,Inc.,were used as starting materials to synthesize the S-rich(x<0.40)and As-rich(x>0.40)bulk As x S1Àx glasses.All weighings were performed in a pure and dry N2ambient.The starting materials sealed in evacuated(5Â10À7Torr)quartz tubings were slowly reacted by heating to700 C,and melts homogenized by continuous rocking in a furnace for48h.Melt temperatures were then slowly lowered to T‘þ50 C and melts equilibrated for several hours before a water quench.Glass transition temperatures T g(x)were measured using a model2920temperature-modulated DSC instrument from TA Instruments,at a3 CÀ1min scan rate(heating followed by cooling)and a1 C per100s modulation rate.T g(x)trends show an increase with increasing As content of the glasses at low x and a global maximum near x¼0.40,as shownin figure 1.The observed T g (x )trend closely parallels those of T ‘(x )(figure 1).Furthermore,T g (x )of a stoichiometric glass,melt quenched from T q ¼550 C (figure 1,open circle)is found to be 6 C lower than that of a sample melt quenched from T q ¼350 C (figure 1,full circles).Raman scattering excited by 6mW of the 647.1nm radiation from a Kr-ion laser,focused to a 50m m spot size,was studied in a back-scattering geometry.The scattered radiation was analysed using a triple-monochromator system (model T64000from Instruments SA,Inc.)and a charge-coupled device detector.Figure 2(a )shows Raman spectra of As 2S 3glass samples synthesized at three different T q values.The scattering strengths of the three modes labelled R in the 150cm À1< <250cm À1range are found to increase steadily with increasing T q .Furthermore,in the main band centred near 340cm À1,a feature near 360cm À1becomes more conspicuous with increasing T q .This group of modes labelled R in the glasses represent the realgar-like nanophase which consists of As 4S 4molecules.Spectra of glasses near x ¼0.40are shown in figure 2(b ).Of special interest is the R mode at 190cm À1,which is not observed at x ¼0.35(see inset of figure 2(b ))but first appears near x ¼0.38and is found to grow monotonically in scattering strength with increasing x thereafter.Figure 3compares Raman spectra of orpiment and realgar (b -As 4S 4)with those of glasses with x ¼0.40and 0.44.The realgar sample was synthesized by annealing an As 4S 4melt for 72h at T ‘¼25 C followed by an air quench,and the nature of the crystalline phase (b -As 4S 4)ascertained by powder X-ray diffraction measurements.The Raman spectra of these crystalline phases are in excellent accord with earlier Intrinsic nanoscale phase separation of bulk As 2S 3glass2943Figure positional trends in glass transition temperature T g and liquidus temperatureT l showing a global maximum near x ¼0.40in the titled binary.The inset shows the modulated DSC traces of the reversing heat flow (Rev.H.F.)and the non-reversing heat flow (Non-Rev.H.F.)of a sample at x ¼0.10with T g ¼38 C and the S polymerization transition temperature T near 140 C.reports (Kobliska and Solin 1973,Ward 1968,Miniz-Miranda et al .1996).Figure 3reveals that the narrow set of modes labelled R and observed in realgar have close parallels in the spectra of the glasses.On the other hand,the sharp modes seen in orpiment and labelled O,also have close parallels in the spectra of the glasses,except that they are blue-shifted by about 18cm À1and significantly broadened in the dis-ordered phase.}3.Discussion3.1.Raman mode assignmentsOur interpretation of these Raman results is as follows.The set of sharp modes labelled R in the glasses are traced to the presence of As 4S 4monomers in the glasses.These intracage bond-stretching mode frequencies in realgar and those in the glasses2944 D.G.Georgiev et al.Figure 2.(a )Raman scattering of As 2S 3glass samples quenched from the indicated melttemperatures T q showing growth in scattering of the modes labelled R with T q (a.u.,arbitrary units).The inset shows the reduction in T g from 214to 206 C when T q is increased from 350to 550 C (Rev.H.F.,reversing heat flow).(b )Raman scattering in binary As x S 1Àx glasses at indicated x showing the onset of the R modes at x ¼0.38(a.u.,arbitrary units).The inset shows a magnified view of the R modes.The mode at 185cm À1at the arrow location onsets near x ¼0.38.are the same (within Æ5cm À1)because the cages are largely decoupled from the host networks.The R modes in glasses are broadened in relation to those in the crystal because of the presence of packing stresses in the disordered phase.The modes labelled O in the glasses are identified with pyramidal As(S 1/2)3units that probably form rings whose size is less certain.The 18cm À1blue shift of the O modes between orpiment and the glasses reflects a transfer of bonding strength from interlayer (van der Waals)interactions to intralayer (covalent)interactions due to loss in planarity of the layers in the glasses.A parallel example occurs in Se,where the bond stretching mode at 233cm À1in trigonal Se is known to upshift to 250cm À1in Se glass due to the more molecular nature of chains in the disordered phase (Zallen and Lucovsky 1976).The O modes are broadened in glasses because As(S 1/2)3units are optimally constrained and can therefore distort,in sharp contrast with modes of Ge(S 1/2)4tetrahedra that continue to be narrow in glasses because of the overconstrained nature of tetrahedra,and therefore less amenable to distortion.3.2.Density functional theory calculations on clustersThe strong similarity between the As 0.44S 0.56glass and crystalline As 4S 4spectra (i.e.c-As 0.5S 0.5or c-As 2S 2)shown in figure 3is compelling evidence for the forma-tion of As 4S 4monomer units in the glass.To support this conclusion further,we have performed first-principles calculations based on the density functional theory (DFT).Specifically,we investigate two cluster models that feature As–As bonds Intrinsic nanoscale phase separation of bulk As 2S 3glass2945Figure 3.Raman scattering in the two crystalline phases,orpiment (crystalline As 2S 3)andrealgar (b -As 4S 4)compared with the line shapes observed in the two indicated glasses at x ¼0.44and 0.40(a.u.,arbitrary units).Features labelled R in the glasses are identified with modes of realgar,while features labelled O in the glasses are identified with modes of orpiment.The orpiment modes in the glasses are blue-shifted by 18cm À1and broadened in relation to the crystalline phase.See text for details.incorporated in different ways into the glass network.The first model features the cage-like As 4S 4unit (figure 4)that forms the basic building block of realgar.Here,the two As–As bonds are connected by four bridging S atoms to form a closed monomer unit that satisfies the valence requirements of all the atoms.In the second model,we open the monomer cage and add two more S atoms.The As dimers still share two bridging S atoms,but the bonds to the remaining S atoms mimic connec-tions to the glass network.H atoms are used to maintain twofold coordination of the outer S atoms in this model.The electronegativity of H (2.1)is similar to that of As (2.0),so that no large unphysical charge transfers are expected owing to the S–H bonds.The DFT calculations were carried out using a Gaussian-orbital-based methodology that has been discussed in detail elsewhere (Pederson and Jackson 1990).Pseudopotentials are used for the heavy atoms,while the H atoms are treated in an all-electron manner (Briley et al .1998).The cluster models are first relaxed to a local minimum-energy configuration.The full vibrational spectrum (including frequencies and mode eigenvectors)of each model is then calculated by diagonalizing the appropriate force constant matrix.Finally,the Raman activities associated with each vibrational mode are calculated directly using the DFT (Jackson 2000),allowing us to compare and contrast the Raman signature of the two models.In table 1we present results for the two models shown in figure 4.The table lists all the modes in both clusters that have significant total Raman intensity in the frequency range 170–400cm À1.The Raman-active modes for both models can be grouped into a low-frequency set (180–230cm À1)and a high-frequency set (350–390cm À1).Analysis of the mode eigenvectors shows that the low-frequency2946 D.G.Georgiev et al.Figure 4.Cluster models of As 4S 4in monomeric and polymeric forms.The dark atoms areAs,and the light atoms are S.H atoms are used to maintain twofold coordination of S atoms in the network model.Intrinsic nanoscale phase separation of bulk As2S3glass2947 Table1.Raman-active modes for two cluster models featuring As–Asbonds.Thefirst column identifies the model(seefigure4).Thesecond column gives the frequencies of the Raman-active modes.The third and fourth columns give the total Raman intensities anddepolarization ratios I HV/I VV of the modes respectively.Cluster!(cmÀ1)I Ram(A4amuÀ1)Monomer18313.10.16221 6.70.063558.20.7537149.40.01 Network195 6.40.14206 6.00.0322711.80.2435118.00.3336932.10.0237133.20.08388 6.90.75 modes correspond mainly to As–As bond stretches,with some admixture of As–S bond bending.The high frequency modes correspond to As–S bond stretches.The calculated Raman-active modes for the monomer model are fully consistent with the assignments made infigures2and3.The low-frequency modes,at183and 221cmÀ1,are an excellent match to the positions of the strong lower-frequency peaks in the crystalline As2S2spectrum and to the corresponding peaks in As0.44S0.56glass spectrum(185cmÀ1and220cmÀ1respectively).In addition,the calculated intensities(13.1versus6.7for the183cmÀ1and221cmÀ1modes respec-tively)agree qualitatively with the observed intensities.This is seen most clearly in the top two curves offigure3.The high-frequency modes of the monomer model,at371and355cmÀ1,are also in good agreement with strong Raman peaks in the crystal and glass spectra at361and346cmÀ1respectively.Again the calculated intensities(49.4and8.2for the371cmÀ1and355cmÀ1modes respectively)are in good agreement with the corresponding observed intensities. The fact that the calculated positions in both cases are stiffer by about10cmÀ1 suggests that this shift is a systematic overestimate of the As–S bond stretch frequency by the DFT.To test this,we also computed the average stretch mode frequency for the AsS3pyramid,the basic building block of As2S3glass.It is reason-able to expect that the calculated stretch mode for the molecular model will be near the centroid of the Raman band of the glass.The calculated symmetric stretch frequency is362cmÀ1,while the centroid in the observed spectrum is near350cmÀ1. Thus the DFT frequency for As–S stretches appears indeed to be somewhat stiffer than observed.We note that the crystalline As2S2Raman spectrum shown infigure3includes a third high-frequency peak near350cmÀ1that has no analogue among the Raman-active monomer frequencies shown in table1.We suspect that this peak corresponds to a doublet of asymmetric As–S stretch modes at343cmÀ1in the isolated monomer spectrum.These modes have very little Raman intensity in the monomer model but have strong infrared intensity.Coupling between monomer units in the crystal may transfer additional Raman intensity to combinations of these modes,giving rise to the additional peak in the crystal spectrum.2948 D.G.Georgiev et al.The results given in table1show clear differences between the monomer and network models.In the low frequency range,the network model has three Raman-active modes,at195,206and227cmÀ1,compared with two modes in the monomer model(at183and221cmÀ1).The network model also has an extra mode in the high-frequency range,at388cmÀ1,that is not present in the monomer spectrum.The remaining high-frequency Raman-active modes are close to corresponding modes in the monomer spectrum.In contrast with the monomer model,the calculated Raman-active modes for the network model do not agree well with the positions and intensities of peaks observed in the crystalline As2S2or As0.44S0.56glass spectra shown infigure3. This is particularly true in the low-frequency part of the spectrum,where the net-work model has modes at195and206cmÀ1.These are stiffer than the observed peak at185cmÀ1.The calculated227cmÀ1mode is also stiffer than the observed peak at220cmÀ1.The calculated relative intensities of the network model modes (6.4,6.0and11.8for the195cmÀ1,206cmÀ1and227cmÀ1modes respectively)also disagree with the intensities of the observed peaks,which suggest that the lower frequency modes should be more intense.Interestingly,the calculated227cmÀ1 mode lies near the observed peak at about232cmÀ1which appears in the As x S1Àx glass spectra,but not in the crystalline As2S2spectrum.As seen in the inset in figure2(b),the232cmÀ1peak appears at around x¼0.35and grows in intensity before the221cmÀ1peak appears at around x¼0.4.A possible interpretation is that, as x increases to0.35,isolated As–As bonds arefirst formed in the glasses.The associated stretch modes are stiffer than those in monomer units and account for the observed Raman peak near232cmÀ1.As the concentration of As–As bonds increases with increasing x,As4S4monomer units begin to form.The Raman sig-nature of the monomers is clearly evident at x¼0.40and the monomer becomes the dominant structural motif for As–As bonds by around x¼0.42.3.3.Broken chemical order in As2S3glassTo test these ideas further,we deconvoluted the observed Raman line shape of the glasses in terms of a superposition of the requisite number of Gaussians,keeping the linewidths,centroids and intensities of each mode unrestricted.The result of the analysis appears infigure5,in which the modes indicated with arrows and labelled R represent those of As4S4monomers while the remaining modes represent those of pyramidally coordinated As(S1/2)3units in orpiment-like sheets.The results offigure5show a systematic growth in the scattering strength of R modes at the expense of O modes as the As content of glasses increases in the0.38<x<0.44 range.The deconvolution of the line shape at x¼2/5,has largely been made possible because of a smooth extrapolation of the Raman results of the As-rich glasses that display sharper features.In the analysis of the broad band centred at340cmÀ1,we found that the relative intensities of the realgar-like modes remain relatively constant within error of measurement.The integrated area under the realgar-like Raman modes normalized to the total area,I R/I(x),is found to increase with increasing x almost linearly once x50.38and,at x¼2/5,the ratio I R/I(x)acquires a value of 0.16(5).We make use of the Raman cross-sections of the R-like and pyramidal As(S1/2)3 modes,given by the cluster DFT calculations,and obtain a fraction of7%of homopolar As bonds in the As2S3glass.The broken chemical order inferredfrom Raman measurements may be compared with a value of 1%established by chemical dissolution of the glass in KOH which selectively extracts As only from As 4S 4units (Kosek et al .1983).The difference between our calculation and the result reported earlier (Kosek et al .1983)is considerable.It is probably due to the over-simplified cluster models and the rather indirect procedure involved in the estimate of the Raman cross-sections.On stoichiometric grounds,the presence of As–As bonds requires S–S bonds to occur in As 2S 3glass.Evidence for S–S bonds in As 2S 3glass emerged independently from 129I Mo ssbauer spectroscopy (Zitkovsky 1989)(see also Zitkovsky and Boolchand (1989)).Here one alloys 129m Te tracer and infers the chemical environ-ment of the chalcogen parent by examining the 129I nuclear hyperfine structure of the former (Boolchand 2000b).The experiments show a bimodal (A,B)site Intrinsic nanoscale phase separation of bulk As 2S 3glass29492950 D.G.Georgiev et al.distribution(Zitkovsky1989,Frumar et al.1997)with I B/I A¼1.60(5).Here,the oversized129m Te tracer segregates to surfaces of large S-rich orpiment-like clusters to populate selectively the roomy and chemically disordered S–S edge sites(Phillips et al.1980y,Zitkovsky1989)(B)over the S-bridging cluster interior(A)sites.The result is a nearly seventyfold enhancement of the B over the A site population (I B>I A)which constitutes evidence for existence of internal surfaces dressing the S-rich cluster.In a homogeneous continuous random network model of As2S3glass, such a large population enhancement I B>I A would be precluded.Furthermore,the present Raman results(figure2(a))show that the degree of nanoscale phase separa-tion in As2S3glass increases with increasing T q as also noted in a previous study (Mamedov et al.1998).The free energy of the phase separated liquid (F¼UÀTSþPV)is apparently lowered by the creation of internal surfaces that provide an additional source of enthalpy U,that is van der Waals bonding and entropy S,particularly if the clusters are small and can move,to overwhelm the endothermic reaction(Pauling1960).2ðAsÀSÞ!ðAsÀAsÞþðSÀSÞÀ11:5kcal molÀ1ð1Þand to promote phase separation as T q is increased.Unlike Raman scattering,75As nuclear quadrupole resonance(NQR)lacks the sensitivity(Taylor et al.1998)y to detect the less than2%concentration of As–As bonds in As2S3glass.Furthermore,since the size of the S-rich clusters is of the order of the wavelength of visible light(as revealed by absorption band tails (Tanaka2001)),NQR can only probe the majority(greater than98%)As sites in the S-rich cluster interior,which are found to be orpiment like,as confirmed by the measured NQR parameters(Taylor et al.1998).3.4.Broken chemical order and T g maximumThe Pauling(1960)single bond strength of a S–S bond of50.9kcal molÀ1exceeds that of an As–S bond of47.25kcal molÀ1.The increase in T g upon alloying As in a S glass is thus qualitatively incompatible with chemical bond strength arguments (Tichy and Ticha1995)that would require T g(x)to decrease with increasing x in the present case.T g reflects the global connectivity of a glass network with differences in covalent bond strengths playing little role,if any,as elucidated by stochastic agglomeration theory(Micoulaut1998)(see also Kerner and Micoulaut(1997)). According to this theory the increase in T g upon alloying As or Ge in a Se glass reflects the increased cross-linking or connectivity of the alloyed network.The global maximum in T g and also of liquidus T‘at the stoichiometric compositions in GeSe2 (Bresser et al.1981)(see also Hazle and Grasseli(1986)and Cai and Boolchand (2002))and As2Se3(Georgiev et al.2000)glasses is a direct consequence of nanoscale phase separation of the glass and the liquid respectively.In these systems,nanoscale phase separation effects are traced respectively to the fact that Ge–Ge bonds and y The surface reconstruction principle proposed by Phillips et al.(1980)has been taken literally by Taylor et al.(1998).If the S-rich cluster size exceeds100nm,then the edge to cluster interior As site concentration ratio becomes less than2%,and NQR measurements will be unable to probe the cluster edge As sites.Intrinsic nanoscale phase separation of bulk As2S3glass2951 As–As bonds do not form part of the backbone once the cation concentration approaches the stoichiometric composition but nucleate separate clusters,as revealed by Raman and Mo ssbauer spectroscopic measurements.The lowering of T‘for off-stoichiometric compositions is a natural consequence of the Born(1939) elastic shear instability(see also Jin et al.(2001))owing to the presence of internal surfaces in corresponding crystals.The reduction in T g of an As2S3glass with increasing T q reflects the loss in global connectivity as the concentration of As4S4molecules increases(figure2(a))and internal surfaces emerge.Infigure1,the sign change in the slope d T g/d x,from a positive value at x¼0.35to a negative value at x¼0.42,reflects the bonding role of As atoms,which serve to increase cross-linking of a S-rich(x¼0.35)network with the free S,but to depolymerize the network of an As-rich glass(x¼0.42)by clustering it.The present results thus form part of a general trend noted in the chalcogenides and has been discussed in more detail in recent reviews(Bresser et al.1981,Boolchand et al.2002).3.5.Broken chemical order and non-Arrhenius temperature variation in viscosityA count of Lagrangian bonding constraints(Georgiev et al.2000)y shows that a network of pyramidally coordinated As(S1/2)3units is optimally constrained,that is three constraints per atom.SiO2glass,composed of a network of tetrahedral building blocks,is also optimally constrained,that is n c¼3,because the bond-bend-ing constraints associated with bridging oxygen atoms are intrinsically broken (Zhang and Boolchand1994).If As2S3glass in analogy to the optimally(Zhang and Boolchand1994)constrained oxide glasses(SiO2and GeO2)were to also form a continuous random network,liquid viscosities would display a strong behaviour, that is an Arrhenius activation with a low activation energy.Viscosities of As2S3 and As2Se3have been measured(Phillips et al.1980)and in sharp contrast with those of SiO2or GeO2melts show an Arrhenius temperature activation with a higher activation energy than in SiO2and GeO2glass.The results show that both As2S3 and As2Se3are mildly fragile.The nanoscale phase separation of the As2S3network contributes fragility(Angell2000)that results in the observed deviation from strong behaviour for much the same reason that addition of alkali to SiO2disrupts the continuous random network and renders it fragile.}4.ConclusionsRaman line-shape deconvolution of As0.4þy S0.6Ày glasses at y¼0.04,when extended to y¼0,show that the stoichiometric As2S3glass is mildly nanoscale phase separated into small As-rich and large S-rich clusters.These results provide a structure basis to understand the local maximum in T g,the broken chemical order inferred in earlier129I Mo ssbauer spectroscopy measurements,weak optical absorp-tion tails and the non-Arrhenius temperature activation of As2S3liquid viscosities and are parallel to results found on other stoichiometric chalcogenide glasses(GeSe2: Boolchand and Bresser2000,Cai and Boolchand2002;As2Se3:Zitkovsky and Boolchand1989,Georgiev et al.2000).y For an As(S1/2)3pyramidal unit, 2.5n c¼[n a(As)þn b(As)]þ1.5[n a(S)þn b(S)]¼(1.5þ3)þ1.5(2)¼7.5or n c¼3.Here n a¼r/2and n b¼2rÀ3,and r¼3for As and r¼2 for S;n c is the count of bond-stretching(a)and bond-bending(b)constraints per atom.。