【精品】SAE paper 外文原文
SAE论文
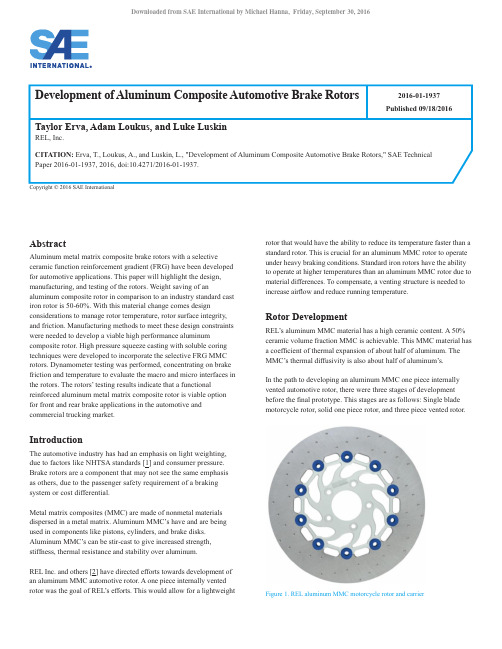
AbstractAluminum metal matrix composite brake rotors with a selective ceramic function reinforcement gradient (FRG) have been developed for automotive applications. This paper will highlight the design, manufacturing, and testing of the rotors. Weight saving of an aluminum composite rotor in comparison to an industry standard cast iron rotor is 50-60%. With this material change comes design considerations to manage rotor temperature, rotor surface integrity, and friction. Manufacturing methods to meet these design constraints were needed to develop a viable high performance aluminum composite rotor. High pressure squeeze casting with soluble coring techniques were developed to incorporate the selective FRG MMC rotors. Dynamometer testing was performed, concentrating on brake friction and temperature to evaluate the macro and micro interfaces in the rotors. The rotors’ testing results indicate that a functional reinforced aluminum metal matrix composite rotor is viable option for front and rear brake applications in the automotive and commercial trucking market.IntroductionThe automotive industry has had an emphasis on light weighting, due to factors like NHTSA standards [1] and consumer pressure. Brake rotors are a component that may not see the same emphasis as others, due to the passenger safety requirement of a braking system or cost differential.Metal matrix composites (MMC) are made of nonmetal materials dispersed in a metal matrix. Aluminum MMC’s have and are being used in components like pistons, cylinders, and brake disks. Aluminum MMC’s can be stir-cast to give increased strength, stiffness, thermal resistance and stability over aluminum.REL Inc. and others [2] have directed efforts towards development of an aluminum MMC automotive rotor. A one piece internally vented rotor was the goal of REL’s efforts. This would allow for a lightweight rotor that would have the ability to reduce its temperature faster than a standard rotor. This is crucial for an aluminum MMC rotor to operate under heavy braking conditions. Standard iron rotors have the ability to operate at higher temperatures than an aluminum MMC rotor due to material differences. To compensate, a venting structure is needed to increase airflow and reduce running temperature.Rotor DevelopmentREL’s aluminum MMC material has a high ceramic content. A 50% ceramic volume fraction MMC is achievable. This MMC material has a coefficient of thermal expansion of about half of aluminum. The MMC’s thermal diffusivity is also about half of aluminum’s.In the path to developing an aluminum MMC one piece internally vented automotive rotor, there were three stages of development before the final prototype. This stages are as follows: Single blademotorcycle rotor, solid one piece rotor, and three piece vented rotor. Figure 1. REL aluminum MMC motorcycle rotor and carrierDevelopment of Aluminum Composite Automotive Brake Rotors2016-01-1937Published 09/18/2016 Taylor Erva, Adam Loukus, and Luke LuskinREL, Inc.CITATION: Erva, T., Loukus, A., and Luskin, L., "Development of Aluminum Composite Automotive Brake Rotors," SAE Technical Paper 2016-01-1937, 2016, doi:10.4271/2016-01-1937.Copyright © 2016 SAE InternationalThe single blade motorcycle rotors are for use by road motorcycles. They are generally thin (≈6mm) and have no center section. The entire blade is composed of aluminum MMC with a function gradient. The rotors are being sold as OE replacements for select street motorcycles.The solid one piece automotive rotor is a single blade rotor with a center hub section that has mounting for an automobile. The rotor is selectively reinforced, with the aluminum MMC section being the friction ring of the rotor. The rotor has external vanes, to help regulatetemperature. The hub and venting section are made of aluminum alloy.Figure 2. REL solid one-piece aluminum MMC automotive rotorThree piece vented automotive rotor is similar to the design of the one piece internally vented automotive rotor. It consists of a center hub section and two rotor blade halves. Each rotor blade half holds half of the internal venting structure. The halves are bolted together and then to the hub. The rotor blade halves and venting sections arealuminum MMC. The hub is made of aluminum alloy.Figure 3. REL vented three-piece aluminum MMC automotive rotorOne Piece Vented Automotive RotorThe automotive rotor has these features: Aluminum with selectively reinforced aluminum MMC sections, one-piece construction, andinternal venting.Figure 4. REL vented one-piece aluminum MMC automotive rotorThe rotor is made up of aluminum alloy and aluminum metal matrix composite material. The ceramic reinforced sections of the rotor are the two braking surfaces. The rotor hub and venting sections are made up of aluminum alloy. The MMC braking surfaces have a function reinforcement gradient (FRG). The ceramic density isradially variable, with an increase in volume fraction from the interior to the perimeter of the preform. This radial change in ceramic volume fraction compensates for the increase in energy and stress on the outer section of the rotor during braking.A computational simulation was done to determine the thermal mechanical performance of a rotor during a stop. Results from the simulation show a difference in stress from the outer edge of the rotor to the inner edge. The pattern of higher stress on the outer edge of the rotor to lower stress on the inner edge of the rotor is followed in a simulation with 0% ceramic content and simulation with 100%ceramic content.Figure 5. Stress simulation with 0% ceramic contentFigure 6. Stress simulation with 100% ceramic contentFigure 7. Stress simulation: rotor edge comparisonTensile testing of aluminum MMC samples was done. Samples of specific ceramic volume fractions were tensile tested and Poisson’s ratio was determined from the results. A trend of decreasing Poisson’s ratio as ceramic volume fraction increases can be observed from the results. With this trend, a gradient of ceramic in the metal matrix composite section of the rotor is used to maintain a rotors integrity during a braking cycle. Higher stress regions have higher ceramic content than lower stress regions of the MMC braking surface.Table 1. Poisson’s ratio of MMCAluminum alloy has a melting temperature of about 550°C lower than iron. However, aluminum MMC has a higher thermalconductivity than iron. These material constraints influenced the design of the rotor. The venting structure in the rotor provides a way for the rotor to regulate its temperature. The curved vanes produce a centrifugal air pump in the rotor when rotated. This airflow created by the pump transfers heat from the rotor to the air. The heated air is then pushed out of the vent. The thermal conductivity of aluminum MMC is higher than iron, so while heat from braking is absorbed faster by an aluminum MMC rotor, heat is dissipated at a faster rate.Venting is crucial to the operation of this rotor.Figure 8. Sectioned internally vented one-piece rotorManufacturingTo manufacture a brake rotor with a high ceramic content and a function reinforcement gradient, high pressure squeeze casting is necessary. The ceramic volume fraction in the in REL rotors cannot be easily achieved by stir cast MMC. To attain the content andgradient of ceramic material, a preform of ceramic material is used. This preform gets infiltrated by aluminum in a high pressure squeeze casting. The infiltration allows the aluminum to form the matrix around the ceramic material.The method of infiltrating ceramic preforms with aluminum gives the ability to have a FRG. The infiltrated preforms are tailored to improve rotor performance. The move away from a stir-cast aluminum MMC method to a preform infiltration method, gives the ability to selectively reinforce the rotor.The aluminum MMC used in the automotive rotor is difficult to machine. Sections of the rotor, venting structure and rotor hub, are not reinforced with ceramic. These sections are not used for braking and do not require improved properties. The majority of the machining required in the non-MMC areas of the rotor.The internal venting in the one piece rotor is not machined andrequires a core. Using high pressure squeeze casting required a high strength core. A salt core had to be developed to withstand thecompressive force required, not be infiltrated, and not lose integrity with temperatures required for casting. The salt core is one piece and holds the negative pattern of the venting structure.The assembly used for casting consists of two ceramic preforms, with a salt core in the middle. The casting assembly is placed in the die and squeeze cast. The aluminum will infiltrate the preforms to create composite material, and be voided by the salt core, creating theventing structure. All other aluminum in the casting will removed orused for rotor hub.Figure 9. REL vented one-piece rotor manufacturing setupTestingThere were three types of testing done with the aluminum MMC rotors. Friction testing was done to determine the ability of a brake rotor to stop with a given set of brake pads. Integrity testing was done to observe the ability of the rotors to withstand braking before failure. Temperature testing was used to evaluate a rotors ability to manage heat added through braking. All tests were done on a brakedynamometer. Thermocouples placed in rotors and pads are used for temperature collection.Friction TestingDifferent brake pads were tested to determine the friction coefficient, brake pad wear and rotor wear when used on aluminum MMC rotors. Non-asbestos organic (NAO) brake pads are preferred for aluminum MMC rotors. Metallic and semi-metallic brake pads are not ideal for the hardness of the composite rotors. All tests shown were completed using the same compound of braking material. It is a NAO brake pad selected for its compatibility with aluminum MMCs.An aluminum MMC rotor was friction tested against a similar cast iron rotor. Both rotors were braked with the same composition of organic material and in the same caliper. The test followed the FMVSS 122 Standard, S7.1 to S7.5. After a brake warming andburnish, the effectiveness tests consisted of six stops from 48.3 km/h (30 mph) and six stops from 96.6 km/h (60 mph) initiated at 65.5°C (150°F) brake temperature. The test concludes with four 128.7 km/h (80 mph) stops and four 160.9 km/h (100 mph) stops in succession. From the test, a brake coefficient of friction for each stop iscalculated. [3]Figure 10. Cast Iron vs Aluminum MMC Friction ResultsThe cast iron and aluminum MMC rotors exhibited similar friction behavior in the test. Through the 30 mph and 60 mph stops, the coefficient of friction for both rotors varied between 0.40 and 0.44. On the 80 mph and 100 mph stops, the MMC rotor saw a steady decrease in its friction coefficient, while the cast iron rotor had a similar decrease but resisted fade better on the final stops.Integrity TestingWith a selectively reinforced rotor, there are two or more different material expansion rates in the rotor as there are two differentmaterials. When subjected to braking, the heat added to the rotor is not constant, linear, or uniform throughout the rotor. Testing on the rotor was done to determine a brake rotors behavior during a heat cycle. Organic brake pads were used. Results of the testing are determined visually. Cracking, separation, degrading, and gougingare all evaluated and compared to other rotors.Figure 11. Integrity testing separation resultsIntegrity testing done consisted of repeated braking without any external cooling. The rotor was stopped from 80 km/h (equivalent speed) at high braking pressure (4500 kPa target) until significant gouging occurred.The results of the integrity testing indicate that the selectivelyreinforced rotor will not fail under temperature change. There was no visible layer separation or significant warping. Gouging occurred at high rotor temperature (≥540°C), exceeding the degradation temperature of the brake pads used.Temperature TestingEvaluating the effectiveness of the venting structure in the rotors was done through temperature testing. The rate of cooling of a vented automotive aluminum MMC rotor was compared against a similar rotor with the venting blocked. Venting was blocked using an epoxy putty. Minimal amounts of epoxy was used, to maintain thesimilarities between the rotors. The test consisted of adding heat to arotor, through a braking cycle, then spinning the rotor at a constant speed (300 rpm). Temperatures are then monitored as the rotor cools down. No external air source was added.Table 2. Vented vs Non-vented cooldown resultsThe comparative results of the cooling of the vented and non-vented rotors showed that the vented rotor will cool faster than a non-vented rotor. This verifies development from solid rotor, to internally vented rotor.In the same manner as the previous test, a vented cast iron rotor was compared to the aluminum MMC vented automotive rotor. Therotation speed was 330 rpm with a cooling wind with of 48.3 km/h.Figure 12. Cast Iron vs Aluminum rotor cooldown resultsThe results of the second temperature test shows the difference in the cooling rates between cast iron and aluminum MMC. The aluminum MMC cooled to 100°C in about half of the time that the cast iron rotor did. This difference in cooling rates allows an aluminum MMCrotor to cool faster than a traditional brake rotor.Figure 13. Aluminum MMC rotor dynamometer testingA third temperature test was run on a dynamometer with two 280mm rotors. One rotor in the test was a prototype aluminum MMC rotor, while the second rotor, like the previous test, was a vented cast iron rotor of similar geometry. The test consisted of ten stops from 80 km/hr equivalent speed and braking with a target maximum brake line pressure of 3500 kPa. There was approximately 20 seconds between each stop. There was an external inline convective airflow produced by an industrial blower that matched the speed of the rotor,simulating the operation of a vehicle. The dynamometer flywheel hada moment of inertia of 78.25 kg*m^2.Figure 14. Aluminum MMC vs cast iron operating temperature testResults from the third temperature test show the operatingtemperatures of a vented aluminum MMC rotor against a comparable cast iron rotor in an aggressive braking test. The MMC rotordisplayed a higher temperature gain per stop than the iron rotor, but also had a greater temperature loss between stops. The cast iron rotor had higher final temperature after the test, but displayed slightlyquicker braking, finishing the test in less time than the MMC rotor.Figure 15. Iron rotor dynamometer testingProductionREL mass produces aluminum MMC motorcycle rotors following the process of squeeze casting aluminum into a ceramic, post cast machining, and heat treatment. The production of a single piecevented aluminum MMC rotor requires an extra component (salt core), two ceramic preforms, and an extra post process (salt removal). Although there are differences the production method of the single blade MMC motorcycle and the process needed for the vented MMC rotor, the method is adaptable to produce the vented MMC rotor.Production costs for a single piece vented aluminum MMC automotive rotor are higher than that of an equivalent cast iron rotor. This is due to a more extensive manufacturing process as well as more expensive materials. When compared to lightweight carbon ceramic rotors, aluminum MMC rotors are significantly cheaper. Market OpportunityNo cost effective one-piece lightweight brake rotor is currently available in the automotive market. Lightweight multi-piece composite rotors are in the high-end auto market. Development is needed to make these components more robust, accepted and cost effective. Though there is widespread demand for this sort of innovation, the expensive composite brake rotors and other lightweight automobile components are only available to the high end and niche applications.The developed lightweight automotive rotor will improve fuel efficiency by allowing for less vehicle weight, lowering the un-sprung weight for better handling and allow for even greater vehicle weight reduction elsewhere by enabling additional complementary weight saving improvements.The commercial potential of the development of a selectively reinforced aluminum composite automobile brake rotor provides a needed solution for the automobile industry. Vehicles that can be targeted for commercialization include lightweight and electric vehicles. Small lightweight vehicle are less demanding of braking systems than full size vehicles. Electric vehicles may have regenerative braking systems that will reduce the amount of braking that is done in the mechanical braking system. In both types of vehicles, electric and lightweight, there is a reduced amount of braking needed compared to a standard vehicle. With limited braking needed, lightweight and electric vehicles are an initial commercialization target for selectively reinforced aluminum composite automobile brake rotors. Beyond this initial target, vehicles from the small car to medium duty truck and military industry can also be targeted for adoption of aluminum MMC rotors. Aluminum MMC Brake DrumsBrake drums made of aluminum metal matrix composite have been developed by Century Inc. The lightweight braking solution can remove 40% of the weight of a similar cast iron brake drum. The brake drums were selectively reinforced and used ceramic preforms and squeeze casting as the method of manufacture. Vehicle and dynamometer testing showed the MMC brake drum either matched or exceeded cast iron drum performance on a 4536 kg axle load. The level of success demonstrated by this brake drum makes it a feasible for the commercial truck and military market. [2] ConclusionAluminum metal matrix composite brake rotors with a selective ceramic function reinforcement gradient have been developed to supplement the demand for light weighting in the auto industry. The development moved from proven single blade motorcycle rotor, to a solid one piece automotive rotor, an internally vented three piece automotive rotor, and finally a one piece internally vented automotive rotor. The intention of development was to produce a lightweight rotor that can be substituted for a standard cast iron rotor. The one piece internally vented rotor has a ceramic function reinforcement gradient, which tailors the MMC rotor sections to a braking cycle, as well as internal venting that will remove heat in the rotor.Rotor development also include development of manufacturing strategies to complement the rotor demands. To be selectively reinforced with a ceramic FRG, the casting method of ceramic preforms and high pressure die casting had to be optimized. Incorporation of an internal venting structure required the development of a large, high strength salt casting core. Dynamometer testing of the aluminum MMC rotor consisted of friction, integrity, and temperature testing. Friction results show that an aluminum MMC rotor and cast iron rotor have similar friction capabilities when using an organic brake pad. Integrity testing results prove that a selectively reinforced rotor can operate without failure at normal braking temperatures. Temperature testing verified the effectiveness of a venting structure as well as the faster cooling rate of an aluminum MMC rotor over a cast iron rotor. The testing results indicate that a functional reinforced aluminum metal matrix composite rotor is viable option for front and rear brake applications in the automotive market.Noise and rusting of rotors was observed during comparison testing of between cast iron and aluminum MMC rotors. The MMC rotors made considerably less noise than the cast iron rotors. While the iron rotors would squeak during braking, the MMC rotors would make a much softer light grinding sound. During storage of rotors, the iron rotors would build an oxidation layer that would be removed before braking. The aluminum MMC rotors did not have a need for oxidation to be removed.This development has been able to prove out the performance and manufacturing of a single piece vented aluminum automotive MMC rotor. Optimization of the rotor and it production process are still necessary for improvement of the product before market introduction. On-vehicle testing is the next step toward pushing development. References1. National Highway Traffic Safety Administration, “CAFÉ - FuelEconomy,” /fuel-economy, accessed Mar.2016.2. Kero, M. and Halonen, A., "Development and Testing ofLightweight Aluminum Composite Brake for Medium to Heavy Duty Vehicles," SAE Technical Paper 2010-01-1705, 2010,doi:10.4271/2010-01-1705.3. National Highway Traffic Safety Administration, Department ofTransportation., “Traffic Safety Administration Laboratory Test Procedure for FMVSS 122,” TP-122-02 August 1, 2006 Contact InformationAdam Loukusadam@Taylor Ervataylor@Luke Luskinluke@REL, Inc.57640 North Eleventh StreetCalumet, MI 49913Phone: 1-906-337-3018rel@Fax: (906) 337-2930AcknowledgementsThe authors would like to thank the Ohio State University for theirwork, support, and results in material testing and computersimulation.Additionally, the authors would like to thank the National ScienceFoundation for their funding of this development effort.The Engineering Meetings Board has approved this paper for publication. It has successfully completed SAE’s peer review process under the supervision of the session organizer. The process requires a minimum of three (3) reviews by industry experts.All rights reserved. No part of this publication may be reproduced, stored in a retrieval system, or transmitted, in any form or by any means, electronic, mechanical, photocopying, recording, or otherwise, without the prior written permission of SAE International.Positions and opinions advanced in this paper are those of the author(s) and not necessarily those of SAE International. The author is solely responsible for the content of the paper.ISSN 0148-7191/2016-01-1937。
SAEpaper
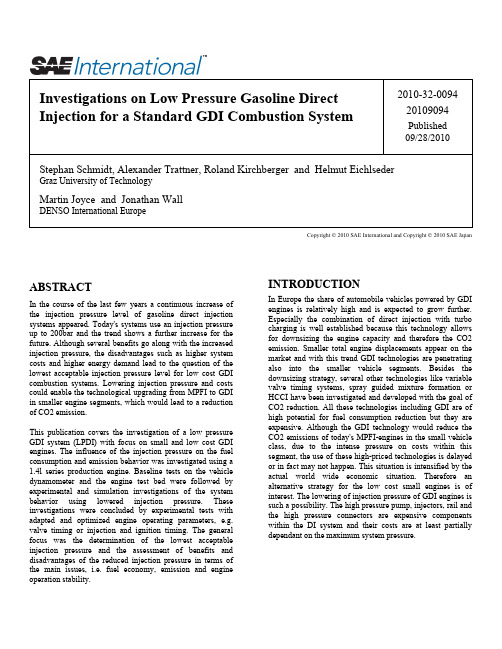
ABSTRACTIn the course of the last few years a continuous increase of the injection pressure level of gasoline direct injection systems appeared. Today's systems use an injection pressure up to 200bar and the trend shows a further increase for the future. Although several benefits go along with the increased injection pressure, the disadvantages such as higher system costs and higher energy demand lead to the question of the lowest acceptable injection pressure level for low cost GDI combustion systems. Lowering injection pressure and costs could enable the technological upgrading from MPFI to GDI in smaller engine segments, which would lead to a reduction of CO2 emission.This publication covers the investigation of a low pressure GDI system (LPDI) with focus on small and low cost GDI engines. The influence of the injection pressure on the fuel consumption and emission behavior was investigated using a 1.4l series production engine. Baseline tests on the vehicle dynamometer and the engine test bed were followed by experimental and simulation investigations of the system behavior using lowered injection pressure. These investigations were concluded by experimental tests with adapted and optimized engine operating parameters, e.g.valve timing or injection and ignition timing. The general focus was the determination of the lowest acceptable injection pressure and the assessment of benefits and disadvantages of the reduced injection pressure in terms of the main issues, i.e. fuel economy, emission and engine operation stability.INTRODUCTIONIn Europe the share of automobile vehicles powered by GDI engines is relatively high and is expected to grow further.Especially the combination of direct injection with turbo charging is well established because this technology allows for downsizing the engine capacity and therefore the CO2emission. Smaller total engine displacements appear on the market and with this trend GDI technologies are penetrating also into the smaller vehicle segments. Besides the downsizing strategy, several other technologies like variable valve timing systems, spray guided mixture formation or HCCI have been investigated and developed with the goal of CO2 reduction. All these technologies including GDI are of high potential for fuel consumption reduction but they are expensive. Although the GDI technology would reduce the CO2 emissions of today's MPFI-engines in the small vehicle class, due to the intense pressure on costs within this segment, the use of these high-priced technologies is delayed or in fact may not happen. This situation is intensified by the actual world wide economic situation. Therefore an alternative strategy for the low cost small engines is of interest. The lowering of injection pressure of GDI engines is such a possibility. The high pressure pump, injectors, rail and the high pressure connectors are expensive components within the DI system and their costs are at least partiallydependant on the maximum system pressure.Investigations on Low Pressure Gasoline Direct Injection for a Standard GDI Combustion System2010-32-009420109094Published 09/28/2010Stephan Schmidt, Alexander Trattner, Roland Kirchberger and Helmut EichlsederGraz University of TechnologyMartin Joyce and Jonathan WallDENSO International EuropeCopyright © 2010 SAE International and Copyright © 2010 SAE JapanFigure 1. Trend of injection pressureA DI system for smaller vehicle classes using lower injection pressure for direct injection (low pressure direct injection LPDI) could be a competitive alternative to today's MPFI engines if this technology is able to reduce fuel consumption and emission compared to port fuel injection. Compared to the continuous increase of injection pressure during the last years this technology would have to use clearly lowered injection pressure (see Figure 1). The purpose of this study is to investigate which level of fuel consumption and emission could be reached when lowering the injection pressure of a standard automotive GDI system. The experimental investigations have been carried out using the standard calibration settings as well as using adapted engine parameters.In the following, the experimental results of the investigated LPDI system with standard automotive hardware are presented. In the first section the requirements and boundary conditions of the investigation are illustrated. Afterwards the results concerning the influence of injection pressure on fuel consumption, emission and combustion behavior are presented, concluding with a short summary. COMBUSTION SYSTEM CONCEPT -REQUIREMENTS / BOUNDARY CONDITIONSBASIC ENGINEThe investigations have been performed on a 1.4l series production engine. The engine is turbo charged and uses high pressure direct injection with a homogenous combustion concept (λ=1). It is a downsized engine with good driving performance, excellent emission behaviour and low fuel consumption. The engine data is shown in Table 1.Table 1. Engine data BOUNDARY CONDITIONSIn order to investigate to lowest injection pressure which results in emission and fuel consumption within acceptable limits with unchanged engine components the present standard engine hardware was used: This includes: solenoid injector with 6-jets, side-positioned injector, standard high pressure pump, intake and exhaust cam and cylinder head. The investigations with reduced pressure have been performed with the series production calibration of the basic engine and an improved calibration setting for low pressure operation.REQUIREMENTSAs the target vehicle class of a LPDI system uses MPFI engines, the requirements and the target values which would define the operation of the technology as potentially acceptable were defined with respect to these engines. In order to ease the assessment of the LPDI technology and to facilitate an evaluation, these values have been defined using an acceptable deterioration of emission and fuel consumption compared to the basic engine with high pressure GDI; not an improvement from an MPFI engine, because such an engine was not tested. These base results have been derived from several emission and fuel consumption tests with the series vehicle on the chassis dynamometer and tests on the engine test bed (Figure 2). The limits have been defined at levels that would be an improvement compared to MPFI technology in series production and should be understood as a “tool” for assessing the value of an LPDI system. Of course, each OEMmight set such limits differently based on their own particularneeds.Figure 2. Tests on chassis dyno and engine test bed The basic engine with HPDI is certified according to EURO IV and reaches 35-45 percent of the legislation limits. The characteristics of HC, CO and NOx in cold NEDC are shownin Figure 3.Figure 3. Emissions in NEDC of basic engine w. HPDI Tail pipe emissions have been measured on the chassis dynamometer and engine out emissions on the engine test bed (Figure 2). Averaged values of fuel consumption and emission and their respective frequency in the engine operating range have been evaluated for the NEDC. This enables the analysis of emission and fuel consumption relevant operation points for the emission test cycle and for real-world driving condition. Figure 4 shows the frequency of operation occurrence in the NEDC, this figur indicates the most operated BMEP and engine speed points during NEDC.For most part of the NEDC the engine is operated with an engine speed beneath 2500min-1 and a BMEP beneath 7bar.The most frequent operation point is 2250min-1 and 3.5barBMEP.Figure 4. Frequency of operation occurrence in NEDC Out of this operation behaviour a fundamental philosophy was derived. The complete engine operation area (max. 18bar BMEP / max 6000min-1) was divided into two areas. An emission cycle relevant area (BMEP 0-8bar / engine speed 700-3250min-1) and a relevant real-world driving area (remaining operation area) were defined. Additionally different criteria have been defined for cold and warm engine operation in the emission cycle relevant area. The criteria define limits for acceptable degradations of engine out emissions, fuel consumption, soot and COVTMEP compared to the values of the basic HPDI engine. In the emission cycle relevant area a deterioration of 5% in cold operation (defined as coolant temperature 40°C) and 10% in warm operation (defined as coolant temperature 95°C) for the engine out emissions has been defined as acceptable. A fuel consumption degradation of 1% in NEDC has been defined as acceptable. For the real-world driving area an increase of 3% fuel consumption and 20% of engine out emissions are considered acceptable for this research study.INFLUENCE OF INJECTION PRESSURE ON COMBUSTION SYSTEMSPRAY BREAK UPThe quality of mixture formation is highly depending on the spray breakup which in turn is strongly influenced by the injection pressure [1]. Spray measurements with a high speed camera have been performed for the adjustment of CFD-models and for a detailed understanding of the quality of the spray breakup at different injection pressure. At high injection pressure the jets have higher velocity than at low injection pressure (Figure 7). This high relative velocity between spray and air results into a faster atomization and better evaporation of the 100bar spray compared to the 50 or 20bar spray (Figure 5, Figure 6).Figure 5. Spray breakup depending on injection pressure(side view / grey shade) [comp. to 1]Figure 6. Spray breakup depending on injection pressure(top view / real picture) [comp. to 1]Figure 7. Exit velocity of spray depending on pressure Due to the different exit velocity and spray breakup the penetration depth changes significantly with the injection pressure (Figure 8). A different mixture formation results from the changed spray breakup and also from the changed spatial fuel concentration distribution. This already indicates that an adjusted calibration for low pressure could beadvantageous.Figure 8. Penetration depth depending on inj. pressureThe change of the primary breakup due to injection pressure variation can be described with the correlation of Ohnesorge and Schneider as shown in Figure 9. At 100bar the spray breakup happens between the atomization and 2nd wind-induced regime. At 20bar the primary breakup is shifted towards the 1st wind-induced regime. Bigger droplet size is the consequence. Equations of Reynolds-, Weber- and theOhnesorge numbers can be found in the appendix.Figure 9. Primary spray breakup [2]The secondary breakup can be described with the correlation of Weber-Nichols. Also the secondary break up is significantly changed with different injection pressure (Figure10) and again bigger droplets are generated.Figure 10. Secondary spray breakup [3]The tested series production injector is designed to work typically with pressures around 100bar. At low injection pressure the closing behaviour is changed and it is assumed that the needle closes more slowly. Certainly large, slowdroplets are the observed (Figure 11).Figure 11. Injector closing at different rail pressure Due to changed spray breakup and the closing behaviour of the injector, the low injection pressure results in larger and slower droplets. This affects the mixture formation negatively. Possible improvements could be: more and smaller holes, an adapted closing and higher charge motion and turbulence in the cylinder. These changes have not been investigated during this study.MECHANICAL LOSSESTheoretical considerations indicate a positive influence of a lowered injection pressure on the mechanical loss. For a quantification of this influence the series production high pressure pump was driven using a modified setup. The mass flow controlled pump is mounted on the cylinder head and driven by the intake cam shaft. In the series production setup,only the actually required fuel flow is compressed (Figure 12). During the tests with changed injection pressure the pump was operated with full volume flow. The rail pressure was controlled by a bypass valve and the excessive fuel wasdelivered back to the pump (Figure 12).Figure 12. Mass flow controlled and full mass flow highpressure pumpThis experimental method of pressure control leads to a lower energy demand of the flow controlled pump compared to the full flow pump whereas the theoretical pump work only depends on the delivered volume and the compressedpressure (Equation 1).Equation 1: Theoretical pump workAt constant volume flow the effective pump work is nearly linearly depending on the difference outlet-inlet pressure.Figure 13 shows the total FMEP of the series production engine at 2000min-1/ 2bar BMEP for different injection pressure and the fuel pump corresponding shares. The orange triangle displays the FMEP of the volume flow controlled pump using the series production setting. The FMEP of the full flow pump (green) is higher and declines linear with lower pressure. The FMEP of the engine operated at 2000min-1 /2bar with 30bar injection pressure is 10% lower compared to the 100bar operation.This advantage is reduced at higher engine speed. On the one hand the high pressure fuel pump (piston pump) is more efficient at higher engine speed, on the other hand the friction of the engine increases with the engine speed. Due to that the FMEP fraction of the pump is lower at high engine speed(Figure 14).Figure 13. FMEP in correlation with rail pressure At 6000min-1 the reduction of injection pressure from 100bar to 40bar results in an 1% improvement of FMEP.Measurements with the flow controlled pump indicate that the positive influence of lower FMEP due to lower rail pressure will be reduced. An FMEP improvement of 5% at low engine speed / WOT and 0.5% at high engine speed /WOT is possible by using a flow controlled system and low injection pressure.Figure 14. FMEP (total FMEP, and FMEP high pressure fuel pump share at different injection pressures)against engine speed at WOT.COMBUSTION BEHAVIOURGeneral behaviourAs a first step of the investigation the following results were investigated without any changes of the calibration except the injection pressure. Injection timing, ignition timing and cam phasing was set at the original series production values.Generally, all tests were conducted with constant lambda=1.The test bed controls the BMEP and engine speed and the ECU of the engine controls the injection mass in order to reach lambda=1.The spray breakup investigations have shown that the atomization is reduced with lower injection pressure. This causes - among other influences (time for evaporation,temperature, cylinder pressure…) - a mixture formation of poorer quality. Additionally the tested series injectors have a bad closing behaviour when using an injection pressure lower than 50bar. This influences mixture formation negatively and increases especial the hydrocarbon emission. Figure 15exemplarily shows the effects of reduced pressure on fuel consumption, emission and combustion stability. For illustration of the general behaviour, a high load point was chosen due to the stronger impact of injection pressure. In the graph a fuel injection pressure limit is drawn based on the degradation limits explained before. In general, fuel consumption and emissions decline with lower pressure and below 50bar the decline gets steeper. HC emissions are most sensitive on injection pressure. Nevertheless, combustion stability nearly stays constant due to the high turbulence caused by the charge motion from the high tumble intake ports. COVIMEP is within the criteria limits over the whole operating range. Even with 10bar injection pressure verygood COV values are achieved.Figure 15. General behaviour of emission and fuelconsumption at lower injection pressure The heat release is also influenced by the injection pressure.The first phase of the combustion is slower by the use of theLPDI. The residual combustion is very similar to HPDI.Figure 16. Combustion behaviour at 2500/10The increasing smoke level (evaluated by FSN) confirms the bad atomization and mixture formation at lower pressure. The engine temperature, defined by the engine coolant temperature, shows a significant influence. The measurements were performed with 40°C and 95°C coolant temperatures Figure 17 displays the FSN for different injection pressures and engine temperature. In warm condition the FSN is little increased at lower pressure. An acceptable value of ∼0.15 is reached at 50bar. In cold condition the margin for soot emission is around 30bar.Beneath 30bar a very steep increase is evident. At 20bar injection pressure unacceptable FSN values appear.Figure 17. FSN at 2500min-1 / 10bar BMEP fordifferent injection pressure In all load points the indicated efficiency decreases due to worsen mixture formation at low injection pressure. But the friction is positively influenced as shown in Figure 13. The improvement of friction results in little better effective efficiency at part load operation points (Figure 18). At higher load the effective efficiency is reduced because thedegradation of inner efficiency is predominateFigure 18. Efficiency depending on injection pressure2000min-1 / 2bar BMEPInfluence of loadGenerally, the higher load increases the degradation of fuel consumption and emissions based on two physical phenomena with opposite effect. The increasing in-cylinder charge motion is positive, as the higher velocity improves atomization and mixture formation and increases the combustion rate. Concurrently, the higher in-cylinder pressure during injection influences the mixture formation negatively as a higher cylinder pressure worsens the evaporation of gasoline [4]. Figure 19 shows the evaporationof gasoline at various ambient pressures.Figure 19. Evaporation of gasoline depending onambient pressure [5]Figure 20 displays the degradation of HC emissions depending on injection pressure and engine load. At higherengine load the degradation of HC emission is worse.Figure 20. HC emission depending on inj. pressure andengine load Compared to the deterioration of HC emission the CO and NOx emissions are less sensitive on the reduction of injection pressure. But these emissions also become more adversely affected by low injection pressures at higher engine loads.Figure 21 shows the NOx emission compared to HPDI at different injection pressures and engine load. At low load nearly equal NOx emissions are emitted. At high load the NOx emissions become worse with lower pressure. Figure 22shows the CO emission. For low load points the CO emission is reduced or equal at low injection pressure. Higher load points lead to increasing CO-emissions for decreasing injection pressure.Figure 21. NOx emission depending on inj. pressure andengine loadFigure 22. CO emission depending on inj. pressure andengine loadInfluence of engine speedWith higher engine speed, the turbulence increases in the cylinder but concurrently the available time for mixture preparation is shortened. These positive and negative effects almost exactly neutralize each other in case of this engine design and therefore the acceptable rail pressure limit is hardly depending on the engine speed. As the influence of engine speed is very low, the characteristic curves are similar when lowering the rail pressure. Figure 23 displays the HC emission of LPDI compared to HPDI at different enginespeed.Figure 23. HC emission against inj. pressure fordifferent engine speedThe degradation of emissions and BSFC depends on the injection pressure only, being constant for different engine speeds. The time for mixture formation is shortened with higher engine speed, but the high turbulence and tumble level supports mixture formation at high engine speed.Influence of engine temperatureThe engine temperature, described by the two extreme conditions cold coolant temperature (40°C) and warm coolant temperature (95°C), strongly influences the acceptable reduction of rail pressure. With lower coolant temperature the degradation with lower injection pressure gets steeper. A noticeable step in the engine characteristics can be found at ∼70°C for BSFC, HC- and NOx-emissions. Above 70°C a pressure reduction below 30bar is acceptable whereas below 70°C engine temperature the acceptable injection pressure is above 60bar. CO emission shows in some areas lower values with reduced pressure than with high injection pressure. FSN is affected again only in low pressure and temperature regions. COV was not degraded even at the lowesttemperatures and pressures measured.Figure 24. HC emissions depending on inj. pressure atdifferent engine temperatures Accompanying 3D CFD simulations of the mixture formation indicate an influence of wall wetting on the degradation of BSFC and emissions with lower engine temperature. As shown in Figure 25 during injection and mixture formation a small amount of fuel mass is transported to the wall area,causing wall wetting.Figure 25. CFD Simulation of the mixture formationThe top limit of the boiling curve of gasoline is approximately at 220°C. When the wall is hotter than this temperature, a major part of the wall film is vaporized again.With a cold cylinder wall more fuel is deposited in the wall film, leading to increased HC emissions, worsened BSFC and oil dilution. The characteristics of HC emissions and BSFC at different engine temperatures are shown in Figure 24 andFigure 26.Figure 26. BSFC depending on inj. pressure at differentengine temperaturesOIL DILUTIONIn DI engines a little amount of fuel mass transported to the cylinder wall area is not evaporated and therefore can be brought into the oil circuit. The continuous absorption of gasoline into oil is called oil dilution. Due to gasoline's low viscosity, the viscosity of the mixture oil-gasoline decreaseslinear with rising fraction of gasoline (Figure 27).Figure 27. Viscosity of oil-gasoline mixtureIf the fraction of gasoline in oil is too high the tribologic quality of the oil can be affected such that the life time of the engine is reduced. When reducing the fuel injection pressure,cylinder wall wetting can be increased; therefore the process of oil dilution has been investigated via a pre-defined test on engine test bed. Oil samples have been extracted in a defined time span and chemically analyzed regarding the gasoline fraction. The results are shown in Figure 28. In warm condition the injection pressure has no influence on the level of oil dilution. In contrast, in cold condition, injection pressure has a big influence due to the changed evaporation conditions. The cylinder wall is too cold to evaporate the absorbed gasoline. Due to bigger droplets and a changed penetration depth of the injection spray the low injection pressure results into higher oil dilution at cold enginecondition.Figure 28. Oil dilution at different temperatures and inj.pressure Generally the reduced injection pressure leads to similar values of oil dilution in warm condition compared to the high injection pressure, whereas 40% more gasoline in oil is evident in cold condition. The oil dilution tests have been performed at one single operation point (2500min-1 5bar BMEP). It turned out that, at this operation point the engine temperature is a main influencing parameter on oil dilution.Additionally, the oil dilution has big influence on the BSFC.Due to lower viscosity the friction of the engine is lower and BSFC is improved whereas the engine life time is reduced.Although a regeneration of the diluted oil might place via evaporation of the contained gasoline, in the case of this engine the oil does not regenerate at all even in high load operation points. A large portion of gasoline remains in the oil and the effects are permanently measureable. In order to get stable and repeatable results the oil was changed after each cold test.RE-CALIBRATION FOR LOW PRESSUREThe investigations with reduced injection pressure showed a potential of improving the mixture formation and consequently BSFC and emissions at a certain pressure level by adjusting the engine's operating parameters. This assumption is based on the fact, that with lowered injection pressure the spatial distribution of the evaporated fuel in the combustion chamber is changed considerable compared to the original setting. This leads to the possibility for improvement by adjusting the ignition and injection timing as well as the intake cam phasing. The variation was performed by using an autonomous test bench ECU for the engine operation.IGNITION TIMINGBy measuring an ignition timing sweep we can see the trade-off between good fuel consumption and good emission behaviour as showed in Figure 29, were an ignition timing set to ∼30°CA gives the best result for BSFC. As is usual, a compromise has to be chosen for the production setup, but a change of the ignition timing is favourable for the changedinjection pressure.Figure 29. Influence of ignition timing on BSFC, NOxand HC emission in part load 2000/2barINJECTION TIMINGThe quality of mixture formation in a GDI engine is, to a very high extent, determined by the injection timing. Generally,with lower injection pressure the mixture preparation is worse as the fuel droplets tend to be bigger. Therefore the injection has to start earlier in order to extend the time for the spray break up and to allow for a good mixture preparation for satisfying emissions and BSFC. The injection timing has to be tuned for each operating point as different conditions for evaporation occur at different operation points. Figure 30shows the cylinder pressure at different SOI during injection.In an area between 290-320°CA the cylinder pressure is quite low. Due to the physical properties of gasoline the boiling temperature is lower at lower ambient pressure and therefore the condition for evaporation during this time is better.Additionally an increased charge motion improves themixture preparation.Figure 30. SOI variation in part loadAs measurements at part load and cold engine condition show (Figure 31), a variation of the SOI changes the engine out emission as well as the BSFC. For 2500RPM and 5bar BMEP An optimum for BSFC and HC emission with moderate increase of NOx emission can be found using an SOI of330°CAFigure 31. Influence of SOI at 40bar injection pressureon emissions and FC in cold conditionThe improvements by adjusted SOI can be seen not only at part load but also at WOT operation. Figure 32 shows the improvement of emission and fuel consumption at 40bar injection pressure due to ∼40°CA earlier SOI. As the measurements show, the combustion stability is onlymarginally influenced by the adjustment of SOI.Figure 32. Influence of SOI at 2500rpm / 18bar BMEPINTAKE CAM PHASINGThe intake cam shaft position is the main parameter for adjusting the residual gas and fresh gas mass for each combustion cycle and influences the in-cylinder charge motion. During the investigations, the intake cam position showed a major influence on emission and fuel consumption;additionally, a strong interaction with injection timing is evident. Especially at part load, more residual gas showed positive effects on emissions and fuel consumption. The higher residual gas ratio as well as the higher temperature during injection leads to improved vaporisation, reduces HC emission and decreases NOx emission caused by lower combustion temperature. In Figure 33 the effect of the optimized engine parameters SOI and cam phasing is displayed. Compared to the original setting with high injection pressure (HPDI reference value left), the lowered injection pressure leads to increased BSFC HC and NOx emission. When optimizing the cam phasing or cam phasing together with SOI the negative effects of the loweredinjection pressure can be changed into improvements.Figure 33. Influence of cam phasing and different SOIat 2000min-1 / 2bar BMEPDEFINITION OF THE LOWESTACCEPTABLE INJECTION PRESSUREWhen contrasting the measured values for BSFC, emission and operation stability using optimized engine parameters with the acceptable limits as defined before, a necessary minimum injection pressure can be defined for different speed/load areas and engine conditions (hot/cold). For each criterion and engine operation point, a margin in the characteristic engine map can be drawn as shown in Figure 34. The yellow line indicates a constant BSFC deterioration of one percent. The coolant temperature influences the potentially acceptable injection pressure. At low coolant temperature a higher injection pressure is required than inwarmer condition.Figure 34. BSFC increase [%] (compared to the original settings at original pressure) against injection pressureand engine temperature When combining the margins of the single criteria, an area of possible injection pressures is generated for each engine operation point. Figure 35 exemplarily displays the area of potentially acceptable injection pressure of each criterion without the change of calibration for a constant BMEP of 10bar and different engine speeds. The colored lines represent the margin of the area (injection pressure & engine speed) for acceptable operation for the different criterion (BSFC, NOx, CO HC). All injection pressure values above these margins lead to acceptable engine operation. For the BSFC criterion, the margin is defined around 45 bars for all engine speeds, which means the minimum pressure has to be above this limit. Out of these limits an overall limit for the reduced injection pressure can be derived. This limit depends on speed and load as well as on the engine coolant temperature. The HC emission is the most sensitive emission component regarding injection pressure reduction.。
SAE论文

ABSTRACT
Due to the increasing use of Fatty Acid Methyl Esters (FAME) in diesel fuel blends and the sensitivity of the latest Diesel engine technology (higher pressures and temperatures), a reliable method for predicting the thermal stability of biodiesel fuels is required. A laboratory bench test, called MicroCoking test, which usually qualifies engine oils, has been modified in order to be used for biodiesel blended fuels. Experimental conditions, and especially temperature range, were optimized in order to lead to a sensitive and discriminating method to qualify pure biodiesel fuels and Diesel blends containing FAME. INTRODUCTION New Diesel engine technologies impose more and more severe constraints on Diesel fuels: temperature and pressure increase in the injection system, the use of a fifth injector in the exhaust line for after treatment system regeneration, etc. Moreover, the increase of Fatty Acid Methyl Esters (FAME) ratio in Diesel fuel formulation modifies some of its physical and chemical properties such as its behavior at high temperature. These facts could lead to varnish or lacquer deposits on some engine parts, especially on the fuel pump and injectors. Consequently, new methods for predicting thermal stability of Diesel fuels containing biodiesel could be very relevant. Numerous test procedures have been developed to measure oxidative stability of FAME, pure or in blends: EN14112 Rancimat, EN12205 gums at various temperatures etc. Nevertheless, methods focussing on thermal stability, ie at higher temperatures, are rare and not so obvious. The oxidation stability tests cover temperature operating range from moderate temperatures around 40 up to 120°C, under air or oxygen atmosphere. These conditions can be compared to conditions the fuel can be subjected to from its storage until its route in the injection system. Thermal stability tests, at higher temperatures (>120°C) are more representative of phenomena which take place from the injection system through the combustion chamber, until the exhaust line.
学术英语原文3单元
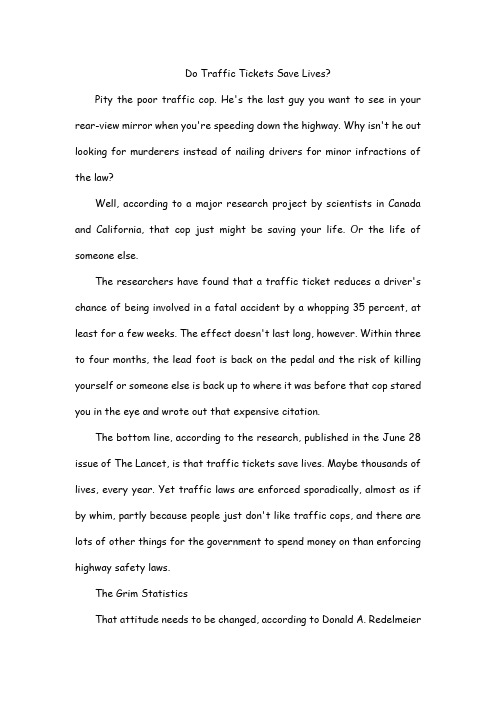
Do Traffic Tickets Save Lives?Pity the poor traffic cop. He's the last guy you want to see in your rear-view mirror when you're speeding down the highway. Why isn't he out looking for murderers instead of nailing drivers for minor infractions of the law?Well, according to a major research project by scientists in Canada and California, that cop just might be saving your life. Or the life of someone else.The researchers have found that a traffic ticket reduces a driver's chance of being involved in a fatal accident by a whopping 35 percent, at least for a few weeks. The effect doesn't last long, however. Within three to four months, the lead foot is back on the pedal and the risk of killing yourself or someone else is back up to where it was before that cop stared you in the eye and wrote out that expensive citation.The bottom line, according to the research, published in the June 28 issue of The Lancet, is that traffic tickets save lives. Maybe thousands of lives, every year. Yet traffic laws are enforced sporadically, almost as if by whim, partly because people just don't like traffic cops, and there are lots of other things for the government to spend money on than enforcing highway safety laws.The Grim StatisticsThat attitude needs to be changed, according to Donald A. Redelmeierof the University of Toronto and Robert J. Tibshirani of Stanford University. Both men are medical researchers, and this isn't the first time they've taken a hard look at highway safety. Their 1998 study caused a stir when they linked cell phone usage to traffic accidents. Now they're back, saying traffic tickets are good for our health.They were prodded into this project by some very grim statistics. Each year, more than a million persons die in traffic accidents worldwide. If that many people died of SARS in a year, the public response would probably border on hysteria, but we have come to accept traffic fatalities as a way of life.In addition, another 25 million people around the world are permanently disabled by traffic accidents, and many of them — as well as the fatalities — are children.Taking It Easy After a Ticket?When Redelmeier and Tibshirani and fellow researcher Leonard Evans set out to see if traffic tickets really do any good, they found an enormous resource in the Canadian province of Ontario. The full driving record of every licensed driver there was made available to them, warts and all, giving the researchers a huge data base of more than 10 million licensed drivers, 8,975 of whom were involved in a fatal accident during the 11-year period covered by the research, from 1988 through 1998."We looked at the month prior to a fatal accident, and the number oftraffic convictions, and then the same month in the year before," says Tibshirani, a statistician. "What we found was that there were fewer tickets in the month before a fatal accident than there were a year before, and that suggests there's a protective effect of having a ticket."In other words, when the number of citations went down, the number of fatal accidents went up the following month, and when the number of tickets went up, the number of fatal accidents dropped the following month. The analysis shows that fatal accidents declined by 35 percent because of citations.Apparently, people just drove more cautiously following a traffic citation, but that only lasted a maximum of four months, the researchers say. After that brief respite, it was back to business as usual for most motorists.Citations’ Effects ConsistentThe scientists also turned up some surprising results."Most of the crashes did not involve alcohol and were not at an intersection," they report in their research paper. Most occurred during the summer months when the streets were dry (65 percent) rather than wet (18 percent) or covered with snow (17 percent).They also found that the "relative risk reduction associated with traffic convictions was remarkably consistent among subgroups of licensed drivers," so the same results apply to women as well as men, regardless ofage, prior driving record, and other personal data.Men, however, were involved in far more fatal accidents than women (73 percent to 27 percent) and the most accident-prone age was between 30 and 50. Alcohol was detected in only 7 percent of the accidents.The researchers also addressed the commonly held belief that traffic citations cause more accidents than they prevent because so many people are killed during police chases. They found that only 24 deaths could be linked to writing citations during the 11-year period. That included 17 suspects, five bystanders and two police officers."The typical suspect who died was a 26-year-old man pursued by police after fleeing a spot check for alcohol or a speeding violation," they report. The two police officers were killed in separate events when they were hit by a car while writing a ticket for another motorist.Who Really Pays?The researchers admit there are a few gaps in their findings. The statistics do not include Ontario drivers who may have been involved in a fatal accident somewhere outside that province. Nor can they say that every traffic ticket leads to a reduction in accidents. But the statistics suggest a correlation between the number of citations and the number of fatalities.They also point out that the innocent are often made to pay the price for careless drivers."Unlike other common diseases, the victims are often young and need significant subsequent care for decades. Most crashes are unintended, unexpected, and could have been prevented by a small difference in driver behavior."So the next time you see that cop in your rear-view mirror, give him, or her, a broad smile.Yeah, right.哎,这可怜的交警。
SAE J1052 机动车辆驾驶员及乘客头部包络及定位(中英文对照)
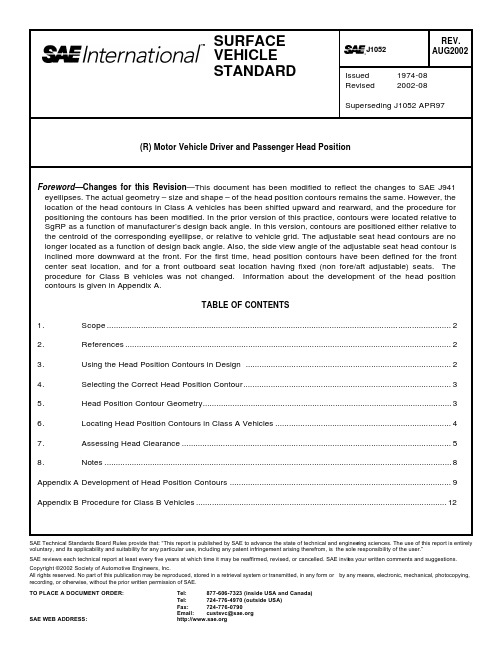
SAE Technical Standards Board Rules provide that: “This report is published by SAE to advance the state of technical and enginee ring sciences. The use of this report is entirely voluntary, and its applicability and suitability for any particular use, including any patent infringement arising therefrom, is the sole responsibility of the user.”SAE reviews each technical report at least every five years at which time it may be reaffirmed, revised, or cancelled. SAE invit e s your written comments and suggestions. Copyright ©2002 Society of Automotive Engineers, Inc.All rights reserved. No part of this publication may be reproduced, stored in a retrieval system or transmitted, in any form or by any means, electronic, mechanical, photocopying, recording, or otherwise, without the prior written permission of SAE.SAE Technical Standards Board Rules provide that: “This report is published by SAE to advance the state of technical and enginee ring sciences. The use of this report is entirely voluntary, and its applicability and suitability for any particular use, including any patent infringement arising therefrom, is the sole responsibility of the user.”SAE reviews each technical report at least every five years at which time it may be reaffirmed, revised, or cancelled. SAE invit e s your written comments and suggestions. Copyright ©2002 Society of Automotive Engineers, Inc.All rights reserved. No part of this publication may be reproduced, stored in a retrieval system or transmitted, in any form or by any means, electronic, mechanical, photocopying, recording, or otherwise, without the prior written permission of SAE.1.Scope—This SAE Recommended Practice describes head position contours and procedures for locating thecontours in a vehicle. Head position contours are useful in establishing accommodation requirements for head space and are required for several measures defined in SAE J1100. Separate contours are defined depending on occupant seat location and the desired percentage (95 and 99) of occupant accommodation.This document is primarily focused on application to Class A vehicles (see SAE J1100), which include most personal-use vehicles (passenger cars, sport utility vehicles, pick-up trucks). A procedure for use in Class B vehicles can be found in Appendix B.2.References2.1Applicable Publications—The following publications form a part of this specification to the extent specifiedherein. Unless otherwise specified, the latest issue of SAE publications shall apply.2.1.1SAE P UBLICATIO NS—Available from SAE, 400 Commonwealth Drive, Warrendale, PA 15096-0001.SAE J941—Motor Vehicle Drivers’ Eye LocationsSAE J1052 MAY87—Motor Vehicle Driver and Passenger Head PositionSAE J1052 APR97—Motor Vehicle Driver and Passenger Head PositionSAE J1100—Motor Vehicle DimensionsSAE J1516—Accommodation Tool Reference PointSAE J1517—Driver Selected Seat PositionSAE Paper 650464—J.F. Meldrum (1965), “Automobile Driver Eye Position,” SAE Mid-Year Meeting, Chicago, ILSAE Paper 720200—D.C. Hammond and R.W. Roe (1972), “Driver Head And Eye Positions,” SAE Annual Congress, Detroit, MISAE Paper 750356—R.W. Roe (1975), “Describing the Driver's Workspace: Eye, Head, Knee, and Seat Positions,” SAE Annual Congress, Detroit, MISAE Paper 852317 (in SAE Special Pub. 712)—N.L. Philippart and T.J. Keuchenmeister (1985),“Describing the Truck Driver Workspace”, SAE Truck & Bus Meeting, Chicago, IL2.1.2UMTRI P UB LICATION—Available from UMTRI, RIPC, 2901 Baxter Road, Ann Arbor, MI 48109-2150. Email:umtridocs@, 734-764-2171.Lee, N.S. and Schneider, L.W. “A Preliminary Investigation of Driver Lean in Late Model Vehicles with Bench and Bucket Seats,” UMTRI Report No. UMTRI-88-49, November 1988ing the Head Position Contours in Design—The following considerations should be kept in mind whenapplying head position contours during design. Head position contours are models that describe occupant head locations for a population, not an individual. In this document, the head contours for Class A vehicles are based on a USA population having an equal number of males and females. Head position contours are constructed as tangent cutoff tools by applying a mean head profile (SAE Paper 750356) to the appropriate tangent cutoff eyellipse. (See Figure 1.) This means that a plane drawn tangent to the surface of a 95 percentile head position contour will result in 95% of the head locations lying on one side of the plane, while 5% will be on the other. It does not mean that the 95th percentile head position contour contains 95 percent of occupant head locations inside its surface.The surface of a head position contour represents the surface of the population of heads with hair. If the top of the 95% head contour is just touching a vehicle surface, this means that 5% of a population consisting of half males/half females would have their head or hair contacting a vehicle surface when sitting in their preferred seating posture. Designers will need to add additional clearance around the head contours in order to prevent occupants’ head or hair from touching vehicle surfaces and structures in normal driving or riding postures.1.范围 —本SAE工业标准描述了头部位置包络以及车辆头部包络定位的设计程序。
外文原文 (2)
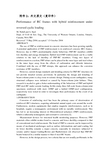
附件2:外文原文(复印件)Performance of RC frames with hybrid reinforcement under reversed cyclic loadingM. Nehdi and A. SaidDept, of Civil & Env. Eng., The University of Western Ontario, London, Ontario, Canada, N6A 5B9Received." 5 May 2004; accepted." 13 October 2004ABSTRACTThe use of FRP as reinforcement in concrete structures has been growing rapidly.A potential application of FRP reinforcement is in reinforced concrete (RC) frames. However, due to FRP's predominantly elastic behaviour, FRP-RC members exhibit low ductility and energy dissipation. Hybrid steel-FRP reinforcement can be a viable solution to the lack of ductility of FRP-RC members. Using two layers of reinforcement in a section, FRP rebars can be placed in the outer layer and steel rebars in the inner layer away from the effects of carbonation and chloride intrusion. Combined with the use of FRP stirrups, this approach can enhance the corrosion resistance of RC members.However, current design standards and detailing criteria for FRP-RC structures do not provide detailed seismic provisions. In particular, the design and detailing of beam-column joints is a key issue in seismic design. During recent earthquakes, many structural collapses were initiated or caused by beam-column joint failures. Thus, research is needed to gain a better understanding of the behaviour of FRP and hybrid FRP-steel-RC under seismic loading. In this study, three full-scale beam-column joint specimens reinforced with steel, GFRP and a hybrid GFRP-steel configuration, respectively were tested in order to investigate their performance in the event of an earthquake.1.INTRODUCTIONCorrosion of reinforcing steel has been the primary cause of deterioration of reinforced (RC) structures, requiring substantial annual repair costs around the world. Furthermore, modem equipments that employ magnetic interferometers, such as in hospitals, require a nonmagnetic environment with no metallic reinforcement. This has led to an increasing interest in fibrereinforced polymers (FRP) reinforcement, which is inherently nonmagnetic and resistant to corrosion [1].Measurement devices for structural health monitoring purposes. However, FRP materials often exhibit weaker bond to concrete and lower ductility compared to that of conventional steel reinforcement. The bond of FRP to concrete can be improved by means of mechanical anchorages such as surface deformations and sand coating, but its lower ductility remains a major concern, especially in structures subjected to seismic and/or impact loading.Brown and Bartholomew [2] observed that FRP-RC beams behaved in a similar manner to that of steel-RC beams.However, in the design process, two criteria that are not usually problematic in thecase of steel reinforcement can govern the design in the case of FRP reinforcement: deflection and ductility. Most FRP materials usually have a significantly lower modulus of elasticity compared to that of steel (except for some new CFRP products) and thus, often generate higher deflections, Furthermore, the predominantly elastic behaviour of FRP results in little warning before a usually sudden and brittle failure. Satisfying deflection and ductility requirements is a challenge in designing FRP-RC structures. Thus, it is recommended that flexural design of FRP-reinforced slabs and beams should aim at over-reinforced sections in order to achieve a concrete compression failure, which usually allows FRP-RC flexural members to exhibit some plastic behaviour before failure [3, 4].In recent years, there has been a growing interest to investigate the performance of mixed steel-FRP as well as steel-free FRP-RC structures. However, research in this area has been generally limited to some beam and column testing.Most of the newly adopted specifications for the design of FRP-reinforced concrete [4-8] are not comprehensive, often do not include detailed seismic provisions, and do not cover hybrid FRP-steel RC systems. Therefore, research is needed to investigate the performance of FlIP and hybrid FRP-steelreinforced concrete frames under reversed cyclic loading in order to form the basis for future design code provisions for FRP-reinforced concrete in seismic zones. In this study, fullscale steel-reinforced, steel-free GFRP-reinforced, and hybrid GFRP-steel-reinforced beam-column joints were tested under reversed cyclic loading; Their behaviour including load-storey drift envelope relationship and energy dissipation were compared and discussed.2. SCOPE OF PREVIOUS WORKThe use of FRP as reinforcement in RC beams was investigated by various researchers. Different permutations of FlIP and steel as longitudinal and transverse reinforcement,respectively were studied [9-11]. Although transverse reinforcement is usually closer to the concrete surface and is therefore more vulnerable to corrosion, limited investigations have been performed on the use of FRP stirrups. The use of FlIP stirrups has been hindered by their limited availability and the fact that a 60% strength reduction factor at bends for various types of FRP is recommended [6]. Also, bending FRP bars to make stirrups typically needs to be performed in production plants with special care and equipment. The use of FRP NEFMAC (New Fiber Composite Material for Reinforcing Concrete) grids can provide a solution to such a problem; a four-ceU unit taken from a NEFMAC grid provides a three-branched stirrup as shown in Fig.Grira and Saatcioglu [12] investigated the use of both steel grids and CFRP grids as stirrups for confinement of concrete columns having longitudinal steel reinforcement. Several grid configurations were used and column specimens were tested under cyclic loading. They concluded that the performance of columns reinforced with CFRP stirrups was comparable to that of columns reinforced with steel stirrups. They also argued that the use of grids whether made of steel or CFRP provides ease of construction and a near-uniform distribution of the confinement pressure along the column, without congesting the reinforcement cage. They reported that the NEFMAC gridbased stirrups failed at their nodes, which is usually thecommon weakness of FRP stirrups. Fukuyama et al. [13] tested a half-scale three-storey AFRP-reinforced concrete frame under quasi-static loading. RA11S aramid-bars were used for the longitudinal reinforcement of columns, RA7S bars were used as flexural reinforcement for beams and slabs, while RA5 bars were used as shear reinforcement. RA11S, RA7S and RA5 are braided bars with cross-sectional areas of 90, 45 and 23 mm 2, respectively. It was argued that frame deformations governed the design. The frame remained elastic up to a drift angle of 1/50 rad, and no substantial decrease in strength took place after rupture of some main beam rebars owing to the high degree of structural indeterminacy of the frame. It was also noted that the rehabilitation of such a frame was easier than that of conventional RC flames since residual deformations were smaller. However, the flame was not tested to collapse and its behaviour under excessive deformations was not reported. Limited research has been performed on hybrid FRP-steel reinforced concrete. Aiello and Ombres [14] tested 6 beams with different configurations of longitudinal reinforcement including steel only, AFRP only, and hybrid AFRP-steel beams, all with steel stirrups. For some of the hybrid specimens, steel was placed with a larger concrete cover to provide extra protection against corrosion. Experimental results showed that such a hybrid system can have lower service deflection and higher ductility at failure than that of the AFRP steel-flee system. Leung and Balendran [15] tested seven RC beams under four point bending. Concrete strength and reinforcement ratios for both steel and GFRP were varied to produce under-reinforced and over-reinforced sections. Steel rebars were placed at 30 mm higher concrete cover compared to that for GFRP rebars. The study showed that for hybrid beams, steel contributed more effectively to the overall behaviour up to yield. Afterwards, the stiffness of yielded steel dropped drastically and the GFRP rebars started to contribute more efficiently to the section resistance. For high strength concrete beams, the increased flexural capacity resulted in shifting the flexural failure into a shear failure.Some research focused on providing ductility to FRP rebars that are manufactured by filament winding or pultrusion. For instance, Tamuzs and Tepfers [16] investigated the properties of a hybrid FRP rod. They used multiple fibre types along with braiding fibre strands around a soft porous core to achieve a more ductile behaviour. The hybrid rods they produced could provide a ductile behaviour, but the difference between the moduli of different fibre strands seemed to cause uneven load transfer, while the compression of the core material caused a reduction of cross-section. A similar study was performed by Bakis et al. [17] who developed pseudo- ductile FRP rods using different types of fibres. The rods behaved in a pseudo-ductile manner when tested under tension, but premature failure took place due to local stress concentrations. Another study performed by Harris et al. [18] developed a ductile hybrid FRP rebar through braiding of various fibres followed by a pultrusion process. Belarbi et al. [19] were also successful in developing composite reinforcing rebars with a relatively more stable stress-strain behaviour in tension and better load-deflection behaviour under four-point bending. However, such rebars are still in early experimental stages and there is not enough data on their field performance, especially under seismic loading.3. EXPERIMENTAL PROGRAMBeam-column joints can be isolated from plane frames at the points of contraflexure. The beam of the current test unit is taken to the mid-span of the bay, while the column is taken from the mid-height of one storey to the mid-height of the next storey.3.1 Steel-reinforced specimen (J1)3.2 GFRP-reinforced specimen (J4)3.3 Hybrid-reinforced specimen (J5)3.4 Test setup and procedure5. DISCUSSIONThe use of FRP as reinforcement in concrete structures has been increasing in popularity, yet various design guidelines and provisions still need to be developed for its safe implementation in large-scale field applications. For instance, the ACI 440.1R-01 identified a wide variety of research issues pertaining to FRP that need to be addressed, some of which are as simple as establishing the statistical variation of the tensile capacity of FRP rebars. Moreover, the contribution of FRP transverse reinforcement to the shear capacity of RC elements needs to be properly evaluated. The lower bond strength of FRP to concrete compared to that of steel imposes difficulties in design, for instance in satisfying rebar development length such as in the case of beam reinforcement anchorage in exterior joints, for which using FRP would require additional embedded length compared to when steel rebars are used. Also the difficulty of manufacturing bends in FRP makes it difficult to adopt this material in reinforcing structurally complicated configurations and needs to be addressed.A major drawback of steel-free FRP-RC systems is their low energy dissipation under earthquake loading, as demonstrated by the performance of the tested FRP-reinforced joint specimen (J4). The energy input from ground motion is equal to the sum of potential, kinematic, damping and hysteretic energy components [22]. The potential and kinematic energy components vanish after the static equilibrium of the structure is reached, while the damping and hysteretic energy components are responsible for energy dissipation. The hysteretic component becomes the major contributor to energy dissipation when significant inelastic deformations take place. Hence, an FRP-reinforced frame may have to be designed with a high damping component so that when added to its relatively limited hysteretic Component, it can dissipate the energy input during an earthquake. Design guidelines for framed RC buildings by the Architecture Institute of Japan, as outlined by Kobayashi et al. [23], entail ensuring seismic performance by overcoming the ductility deficiency of FRP-RC frames. The study recommended the use of the capacity spectrum method. Performance demand and capacity spectra were evaluated and a performance point, where the demand and capacity spectra meet and members are still below their flexural capacity, was defined as the safety limit. This performance-based design approach was successfully applied to the analysis of a 9-floor FRP-RC frame. The study also pointed out the cruciality of damping in FRP-RC structures and recommended the use of vibration control devices.The use of hybrid steel-FRP RC systems could address many of the drawbacks ofsteel-free RC systems. Steel reinforcement can be used in lateral load resisting structural members, which are not usually exposed to aggressive media, while FRP reinforcement can be used in the envelope of the structure to enhance durability. Alternatively, a hybrid reinforcement configuration can make use of the corrodible steel at a thick concrete cover, while the more durable FRP stays at a minimum cover. Thus, the structure can benefit from using such a hybrid reinforcement system to provide both durability (using FRP) and post-peak reserve strength (using steel).The present study focussed only on comparing the behaviour of FRP, hybrid steel-FRP, and steel-reinforced beam-column joints. Full-scale tests on entire FRP and hybrid-reinforced frames need to be performed to assess the progress of failure globally. The results can be used to calibrate numerical models that can be used to simulate the behaviour of multi-storey FRP and hybrid-reinforced frames with high degrees of redundancy, and accordingly predict the progress of failure. Moreover, passive energy dissipation devices can provide a source of energy dissipation for FRP-reinforced frames, which needs further focussed research. Overall, research efforts are still needed to address many questions and uncertainties, and to develop adequate design provisions dedicated to steel-free and hybrid RC systems, before their widespread use in demanding large-scale structural applications becomes feasible and safe in seismic areas.6. CONCLUSIONSAn effort was made to investigate the performance of GFRP and hybrid steel-GFRP-reinforced beam-column joints and to compare their behaviour to that of standard steel-reinforced beam-column joints under reversed quasi- static (cyclic) loading.,the following conclusions can be drawn:The GFRP-reinforced beam-column joint showed very low plasticity features when tested under reversed cyclic loading. This resulted in lower energy dissipation compared to that of the steel and hybrid reinforced specimens.The hybrid GFRP-steel-reinforced beam-colunm joint showed lower stiffness than that of the conventional steel- reinforced beam-column joint, but exhibited higher stiffness than that of the GFRP-reinforced specimen.The GFRP and hybrid-reinforced specimens showed satisfactory drift capacity, assuming a minimum drift requirement of 3% (0.03 rad) as recommended in the literature for ductile RC flame buildings [24].A hybrid RC system could be tailored to provide a range of performance requirements such as durability, stiffness, strength, ductility, etc. A designer may adapt the reinforcement configuration of the hybrid system to accommodate a balance between such design criteria.This study was only focussed on the level of the subassemblage. A more global concept should be adopted in the design of moment-resisting frames. Thorough dynamic analysis of GFRP and hybrid-RC structures should be performed to better assess their capacity in meeting seismic resistance requirements.Design code provisions for the seismic design of RC structures, which have been developed for ductile steel reinforcement, need to be re-evaluated for FRP-RC structures.。
paper制作过程英语作文
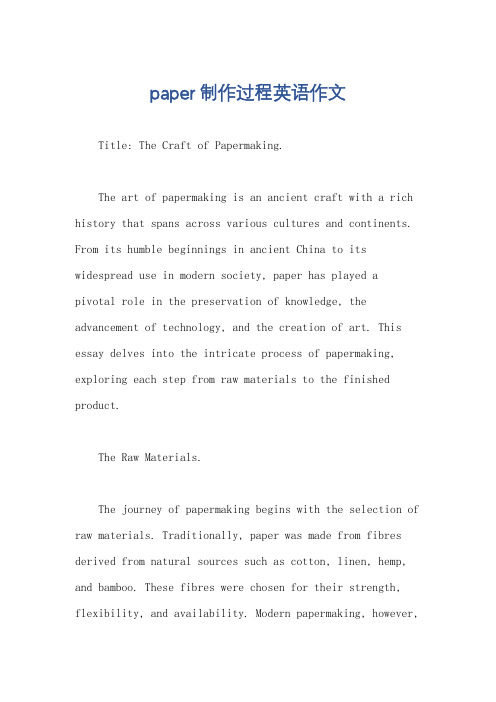
paper制作过程英语作文Title: The Craft of Papermaking.The art of papermaking is an ancient craft with a rich history that spans across various cultures and continents. From its humble beginnings in ancient China to its widespread use in modern society, paper has played apivotal role in the preservation of knowledge, the advancement of technology, and the creation of art. This essay delves into the intricate process of papermaking, exploring each step from raw materials to the finished product.The Raw Materials.The journey of papermaking begins with the selection of raw materials. Traditionally, paper was made from fibres derived from natural sources such as cotton, linen, hemp, and bamboo. These fibres were chosen for their strength, flexibility, and availability. Modern papermaking, however,has expanded to include synthetic fibres and recycled paper, offering a more sustainable and environmentally friendly option.The Soaking and Pulping Process.Once the fibres are selected, they undergo a soaking process where they are immersed in water for several hoursto soften and loosen the fibres. This step is crucial as it prepares the fibres for the next stage, pulping. In the pulping stage, the fibres are mixed with water and chemical agents such as alkali to break down the lignin, a substance that binds the fibres together in their natural state. The resulting pulp is a slurry of fibres ready for the next stage.The Forming Process.The forming stage is where the pulp slurry is transformed into a sheet of paper. This is achieved by pouring the slurry onto a mesh screen or a rotatingcylinder called a Fourdrinier machine. As the water drainsthrough the mesh, the fibres adhere to each other, forming a thin layer of paper. This layer is then gently pressed to remove excess water and consolidate the fibres.The Pressing and Drying Process.After formation, the paper goes through a pressing process where it is compressed between rollers to remove any remaining water and enhance its smoothness. This step is crucial in ensuring the strength and durability of the paper. Following pressing, the paper is dried, either through the application of heat or by allowing it to air-dry. The drying process completes the transformation of the pulp slurry into a solid sheet of paper.The Finishing and Coating.Once dry, the paper can undergo further processing depending on its intended use. This may include cutting the paper to size, applying coatings for smoother surfaces or enhanced durability, and even printing or embossing for decorative purposes. These finishing steps ensure that thepaper is tailored to meet the specific requirements of the end-user.The Evolution of Papermaking.Over the centuries, the art of papermaking has evolved significantly. Ancient methods relied heavily on natural resources and manual labour, resulting in limitedproduction and higher costs. However, with the advent of industrialization and technological advancements, papermaking has become more mechanized and efficient. Today, modern papermaking facilities employ automated machineryand advanced technologies to produce paper in large quantities with consistent quality.The Impact of Papermaking.The impact of papermaking on society is immense. Paper has become a ubiquitous presence in our daily lives,serving as a medium for communication, education, and creativity. It is the backbone of the publishing industry, enabling the dissemination of knowledge and ideas. Fromnewspapers and books to packaging and printing, paper plays a vital role in almost every aspect of modern life.In conclusion, the art of papermaking is a fascinating testament to human ingenuity and craftsmanship. It represents a harmonious blend of natural resources, technology, and human effort, resulting in a product that has profoundly shaped our world. From its humble beginnings to its widespread influence today, the story of papermaking is one that illustrates the transformative power of human creativity and innovation.。
SAE Paper 2011-01-0247_
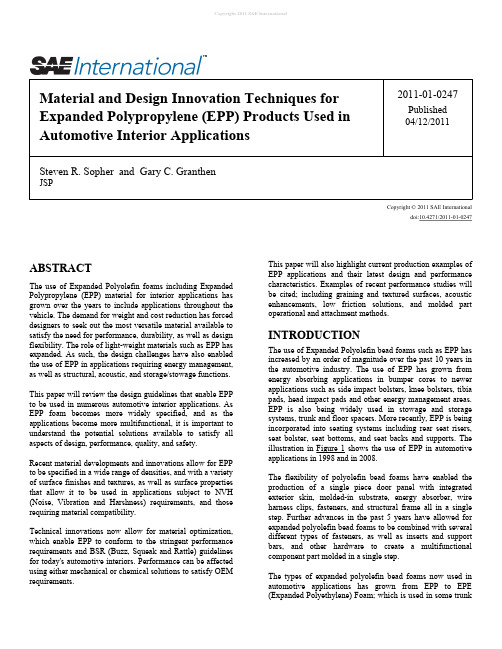
ABSTRACTThe use of Expanded Polyolefin foams including Expanded Polypropylene (EPP) material for interior applications has grown over the years to include applications throughout the vehicle. The demand for weight and cost reduction has forced designers to seek out the most versatile material available to satisfy the need for performance, durability, as well as design flexibility. The role of light-weight materials such as EPP has expanded. As such, the design challenges have also enabled the use of EPP in applications requiring energy management,as well as structural, acoustic, and storage/stowage functions.This paper will review the design guidelines that enable EPP to be used in numerous automotive interior applications. As EPP foam becomes more widely specified, and as the applications become more multifunctional, it is important to understand the potential solutions available to satisfy all aspects of design, performance, quality, and safety.Recent material developments and innovations allow for EPP to be specified in a wide range of densities, and with a variety of surface finishes and textures, as well as surface properties that allow it to be used in applications subject to NVH (Noise, Vibration and Harshness) requirements, and those requiring material compatibility.Technical innovations now allow for material optimization,which enable EPP to conform to the stringent performance requirements and BSR (Buzz, Squeak and Rattle) guidelines for today's automotive interiors. Performance can be affected using either mechanical or chemical solutions to satisfy OEM requirements.This paper will also highlight current production examples of EPP applications and their latest design and performance characteristics. Examples of recent performance studies will be cited; including graining and textured surfaces, acoustic enhancements, low friction solutions, and molded part operational and attachment methods.INTRODUCTIONThe use of Expanded Polyolefin bead foams such as EPP has increased by an order of magnitude over the past 10 years in the automotive industry. The use of EPP has grown from energy absorbing applications in bumper cores to newer applications such as side impact bolsters, knee bolsters, tibia pads, head impact pads and other energy management areas.EPP is also being widely used in stowage and storage systems, trunk and floor spacers. More recently, EPP is being incorporated into seating systems including rear seat risers,seat bolster, seat bottoms, and seat backs and supports. The illustration in Figure 1 shows the use of EPP in automotive applications in 1998 and in 2008.The flexibility of polyolefin bead foams have enabled the production of a single piece door panel with integrated exterior skin, molded-in substrate, energy absorber, wire harness clips, fasteners, and structural frame all in a single step. Further advances in the past 5 years have allowed for expanded polyolefin bead foams to be combined with several different types of fasteners, as well as inserts and support bars, and other hardware to create a multifunctional component part molded in a single step.The types of expanded polyolefin bead foams now used in automotive applications has grown from EPP to EPE(Expanded Polyethylene) Foam; which is used in some trunkMaterial and Design Innovation Techniques for Expanded Polypropylene (EPP) Products Used in Automotive Interior Applications2011-01-0247Published 04/12/2011Steven R. Sopher and Gary C. GranthenJSPCopyright © 2011 SAE Internationaldoi:10.4271/2011-01-0247storage applications. Softer foams like xEPE (Cross-linked Expanded Polyethylene) Foam is also being used to replace soft and semi-rigid PU foams in seat bolsters and headrests. Specialty EPP foams including PEPP (Porous Expanded Polypropylene) Foam, which provides unique energy management properties coupled with unique acoustical benefits such as sound absorption and controlled air flow are also being used. Newer developments in Expanded Polyolefin bead foam technology includes the use of Bio-based foam blends, foams with unique surface resistivity for sensitive electronic packaging as well as foams with unique additives or surface textures used to provide NVH countermeasures such as squeak, and ease of removal. The use of fasteners and integrated support structures both insert molded and secondarily attached is also growing and expanding into multiple applications.DESIGN AND PERFORMANCE INNOVATIONSWith the use of expanded polyolefin foams like EPP continuing to grow, it is important to understand the advantages of EPP and the numerous design options available in the area of performance and cosmetic or aesthetic.One of the features of expanded polyolefin bead foam is the ability of the stress-strain curve to maintain a consistent shape throughout the varying density ranges. The linear correlation between stress-strain and density allows for a specific density to be chosen for each part design. See Figure 2 below for a comparison on [quasi] static stress-strain curves for various EPP densities of 30, 60, 90 and 150 g/l. Expanded polyolefin bead foams like EPP have been modeled over the years and currently provide for a very robust material model for design optimization. The strain rate dependency of expanded polyolefin bead foams allow for predictive modeling for virtually all impact velocities ranging from a quasi-static speed of 10 mm/minute to over 10 m/s (36 kph).One of the benefits of expanded polyolefin bead foam materials like EPP is the ability to match competitive material performance based on the stress-strain characteristics. EPP has replaced a variety of competitive materials throughout the automotive interior. These details of these are shown below in Figure 3:Unlike most PU based materials and other solid resin injection molded materials, both of which are used for support, and energy management, the polyolefin bead foam stress-strain curve does not contain the wide plateau region associated with these types of materials. Figure 4 illustrates a comparison of several types of Polyolefin Bead Foam materials including EPP, PEPP, EPE, xEPE. Note that all curves represent the same molded density; in this case 30 g/l (1.9 pcf). It is important to note that the shape of the stress-strain curve for each material type is similar across all densities, with a corresponding shift upward in Stress as the densities increase.Depending on the specific automotive interior application, different Expanded Polyolefin Bead Foam materials can be specified to meet a specific performance requirement, and in most cases, at a lower density, and henceforth a lower part weight.COMPARISON OF PP & PE BASED FOAM PERFORMANCEWhen comparing the energy management performance of all bead foams, it is important to understand the inherent properties of polyolefin bead foams including those comprising both PP and PE materials. As an expanded bead foam material, EPP offers a good blend of toughness, resilience, impact strength and stress/crack resistance. It offers good low temperature performance, and good chemical resistance. On the other hand, as an expanded bead foam material, EPE (standard and cross-linked) offers excellent resilience, excellent tensile properties, and excellent chemical resistance. It also offers a very low coefficient offriction, Figure 1.which is beneficial for cushioning applications and for housing products in need of abrasion protection. This characteristic of EPE foams is due to inherent nature of PE resin as well as the nature of the EPE foam, which is comprised of very small cells (<100 µm). Both EPP and EPE materials can be optimized for a specific end application based on the selection of the specific base resin.When comparing the relative ‘hardness’ (a.k.a. ‘softness) of various expanded foams, the term Indentation Force Deflection (ILD) is sometime used. This term (found in ASTM D3575) refers to the load a specific foam will manage across a specific surface at a given thickness when compressed 25%. It is an industry term used to characterize relative foam ‘hardness’ for purposes of applications ranging from cushioning, seating, padding, and packaging. Polyolefin Bead Foams can meet specific hardness requirements by specifying a given density.With Expanded Polyolefin Bead Foams gaining wide acceptance within the automotive interior, other methods of characterizing the material ‘hardness’ are being employed. In the case of EPP replacing other semi-rigid materials such as TPE's, TPV's, and TPU based materials, specific material hardness standards are being referenced for comparison. Several industry standards exist including ASTM D2240 and F1957 as well as other OEM and International Standards. These standards include the use of different types of hardness indicators (durometers) which utilize hardness scales such as Shore A, Shore C, Shore D and JIS/Asker C. Each of these scales are referenced for a specific class of materials, including thermoplastics, thermoplastic elastomers, and thermoset rubbers.The most common durometer scale recommended for EPP is the Type C or Asker Type C scale. The chart in Figure 5 below characterizes the hardness scale vs. density for EPP, EPE and xEPE.When replacing these TPE, TPV, and TPU materials with Polyolefin Bead Foams, it is important to understand the specific scale used to compare the materials. While the hardness of EPP encompasses several hardness scales, including Shore A, Shore D and JIS/Asker C, specific densities can be referenced to achieve a specific hardness specification. On the other hand, softer Polyolefin Bead foams like EPE and xEPE fall within the Shore A and JIS/ Asker C scales only. In fact, at the lower end of these scales, softer foams may require the use of a different durometer scale such as Type O, OO or even OOO depending on the application. Figure 6 illustrates the hardness rangesand Figure 2.durometer scales that may be obtained using Polyolefin bead foams such as EPP, EPE and xEPE.SURFACE CHARACTERISTICSWith the use of polyolefin bead foams growing, it has become necessary to improve the surface appearance for applications that are visible (seat base, trunk kits, etc.) to the customer. The use of surface texturing is now commonplace for creating textures and grains on EPP used in some automotive applications. These surface textures can be applied to the mold cavity (Aluminum) and can be created using chemical or laser etching techniques.Surface textures can be used to accomplish a number of things including, camouflaging the part by providing for a more ‘matte’ finish. This has been done on bumper energy absorbers where a section of the part is visible behind the grille, or is visually exposed. Surface textures can be used to provide a more uniform surface finish, and to hide or minimize the ‘beady’ surface appearance sometimes associated with a shape molded expanded bead foam part. It can also be used to providing a more visually appealing surface texture for exposed parts. Finally, the use of surface textures can be used to increase the surface area of the part, and serve to minimize the surface friction of the part, thus preventing the part from ‘squeaking’ when contacted with mismatch surfaces. This can be accomplished on the entire tool, or with local areas of the tool as required by the specific application.While there are virtually unlimited types of surface textures and surface finishes available, it is important to take into consideration the molded density of the Expanded Polyolefin Bead Foam part and the specific depth of the surface texture being specified. While surface textures on injection molded parts can impart a finish with as little as 0.005″ depth, Expanded Polyolefin Bead Foam materials require more depth to achieve adequate texture or grain resolution. In most cases, a surface texture range of 0.025 to 0.040″ is required (depending on molded density) to provide an optimal surface appearance.As the use of Expanded Polyolefin Bead Foams continues to grow, and with the move to further reduce vehicle weight, the trend toward lower and lower molded part densities continues. While materials such as EPP have met the need for reduced weight and optimal performance, it is important to note the long term effect of performance, fit and function when matching EPP with other composite, plastic, or metal materials. The use of surface texturing can serve to minimize surface abrasion, and surface interaction (squeak, vibration, etc.) with these dissimilar surfaces. While there are a number of solutions to reducing these surface interactions including the use of chemical slip additives, tapes, coatings, or internal/ external lubricants, many of these provide only short term protection. Furthermore, it is important to understandthe Figure 3.exact mechanism responsible for the surface interaction before a specific countermeasure is utilized.One of the ways to characterize the potential for a material to interact with dissimilar surfaces is to measure the coefficient of friction. In the case of EPP, the [Kinetic] coefficient of friction increases as the density decreases, which translates to greater potential for surface interaction such as squeak or vibration as the densities are reduced. Figure 7 characterizes the coefficient of friction for EPP at molded densities from 20 to 60 g/l.The chart above in Figure 7 represents a standard tool (polished metal) surface without any surface texture. With the baseline coefficient of friction established for EPP, additional studies were conducted to evaluate the effect of surface texturing the tool. Several surface textures or grains were selected for the study, many of which are currently utilized by the EPP molding community. Figure 8 provides pictures and identifies each of the surface textures selected for the study.The result of the study showed that certain surface textures tend to reduce the coefficient of friction better than others. The study was conducted on 30 g/l (1.9 pcf) EPP on steel using 0.355 psi (2.5 kPa) surface pressure, which has been used as the industry standard for characterizing EPP. The results of the studies are shown below in Figure 9. Note that all surface textures tested provided improved (lower) coefficient of friction results vs. the un-textured (standard molded) surface finish.The results of the study demonstrate that surface texturing by way of altering the molded tool surface can act as an effective countermeasure in reducing the surface interaction (squeak, vibration, etc.) between EPP and other mating components for automotive interior applications. Further studies have indicated that the potential for surface interactions with EPP is minimized with densities > 40 g/l, in which case surface texturing is not necessary other than visual or aesthetic reasons. It should also be noted that a majority of surface interactions that result in minor squeak or vibration can be minimized by optimizing the part dimensions, part datum structure or part installation method. As is the case with most parts, the potential for surface interaction is very design dependent and is contingent on what surfaces or features are subject to interaction, as well as mating part installation, fit and function.RECYCLABILITY AND SUSTAINABILITYExpanded Polypropylene is 100% recyclable and can berecycled from the finished product (post-consumer waste) as Figure 4.well as the in-process scrap. In fact, efforts have been made locally (in Michigan, USA and in Ontario, Canada) to centralize collection of EPP scrap and make use of both manufacturing scrap (from the EPP molders) and End-Of-Life products. It should be noted that molded EPP bumpers can be shredded and remolded by blending virgin EPP with low levels (≤15%) of recycled EPP without compromising the ability to meet the OEM material property requirements.Once collected, the EPP parts are mechanically shredded and/or separated to create rough EPP beads, which are subsequently de-dusted. The EPP steam chest molding equipment and handing system is capable of accommodating a percentage of these recycled beads to be re-introduced into new molded parts. Several studies have been performed on parts produced with this process, and it was noted that certain part aspect and properties can be slightly altered by re-introducing recycled content. As a result eachapplicationFigure 5.Figure 6.should be reviewed to assure that all performance requirements have been satisfied.The base resin utilized to manufacture EPP is a widely used polypropylene copolymer that is suitable for collection and distribution. As a result, scrap EPP parts can be collected, shredded and densified and subsequently 100% recycled by conventional thermoplastic extrusion. The resulting PP pellets can used in any thermoplastic manufacturing process or even introduced into expanded polypropylene bead production.It is important to consider the geographical locations of scrap collection sites, recycling machines, transportation and handling costs designing any new recycling processes, to assure that in addition to its environmental benefit the operation is economically viable.As a measure of the eco-concept potential of EPP, a detailed Life Cycle Analysis was conducted for a specific application, an automotive seat core1. The ARPRO®EPP solution provided an environmental benefit to impact ratio of 12:1 resulting in significant fuel and CO2emissions savings thanks to reduced weight of up to 35% compared to the previous solution used for this application. SUMMARY/CONCLUSIONSThe use of Polyolefin bead foams like EPP in automotive interiors continues to grow. As a result, the marketable features of EPP are also increasing. The ability to mold a wide range of densities, the ability to mold multiple densities in a single part, the ability to produce molded part with integrated inserts and with specific surface finishes are all imperative in order to expand the use of EPP. All of these are indeed possible with EPP. Expanded Polyolefin bead foams like EPP are lightweight, clean material that offers high energy absorbing efficiency, multiple impact performance, design freedom, and an excellent recyclability. With EPP it is possible to realize exceptional cost to performance ratio thanks to the high expansion rate (low density) which can be achieved uniformly throughout the final molded component. The dynamic properties set EPP aside from other foamed plastics, and provide a useful replacement for traditional energy management, structural and storage systems andcomponents.Figure 7.In the global automotive arena, new applications and newgrades of Polyolefin bead foams like EPP are continuouslybeing developed as the material finds new uses where itsproperties offer technical benefits with no environmentalissues.Figure 8. Figure 9.REFERENCES1. JSP Website , Life Cycle Assessment and Physical Properties for Expanded Bead Foam Products; ARPRO EPP, ARPAK EPE, ARPEX xEPE, 20092. Sopher, Steven R., Advanced Development of Molded Expanded Polypropylene and Polyethylene Bead Foam Technology for Energy Absorption. Proceeding of 2005 SPE Annual Technical Conference, May 2005, Boston, MA, USA3. Progelhof, R. C. and Throne, J.L., Polymer Engineering Principles, Carl Hanser Verlag, Munich, 19934. ASTM International, American Society for Testing and Materials, West Conshohocken, PA, USA, 2006-2010.5. Harper, Charles A., Handbook of Plastics, Elastomers and Composites. McGraw-Hill, New York, 2001.ARPRO® EPP is a registered trademark of JSP Licenses LLC ARPAK®EPE is a registered trademark of JSP Licenses LLCARPEX®xEPE is a registered trademark of JSP Licenses LLCCONTACT INFORMATIONSteve Sopher is the Technical Director at JSP. He has over 23 years experience in the plastics industry and over 20 years in the automotive industry. He can be reached at steve.sopher@.DEFINITIONS/ABBREVIATIONS BSRBuzz, Squeak and RattleEPEExpanded PolyethyleneEPPExpanded PolypropyleneNVHNoise, Vibration and HarshnessPEPPPorous Expanded PolypropyleneGary Granthen is a Senior Design and Application Engineer at JSP. He has over 20 years experience in the automotive industry. He can be reached at gary.granthen@ ACKNOWLEDGMENTSThe authors would like to thank their colleagues in the JSP Technical Group and the JSP Design Engineering Group for their help in testing and evaluating the materials outlined in this paper.g/lgrams per liter (equivalent to kg/m3) pcfpounds per cubic foot (lbs/ft3)TPOThermoplastic PolyolefinTPEThermoplastic ElastomerTPVThermoplastic VulcanizateTPUThermoplastic PolyurethanexEPECross-linked Expanded PolyethyleneThe Engineering Meetings Board has approved this paper for publication. It has successfully completed SAE's peer review process under the supervision of the session organizer. This process requires a minimum of three (3) reviews by industry experts.All rights reserved. No part of this publication may be reproduced, stored in a retrieval system, or transmitted, in any form or by any means, electronic, mechanical, photocopying, recording, or otherwise, without the prior written permission of SAE.ISSN 0148-7191Positions and opinions advanced in this paper are those of the author(s) and not necessarily those of SAE. The author is solely responsible for the content of the paper. SAE Customer Service:Tel: 877-606-7323 (inside USA and Canada)Tel: 724-776-4970 (outside USA)Fax: 724-776-0790Email: CustomerService@SAE Web Address: Printed in USA。
SAEJ1681v001
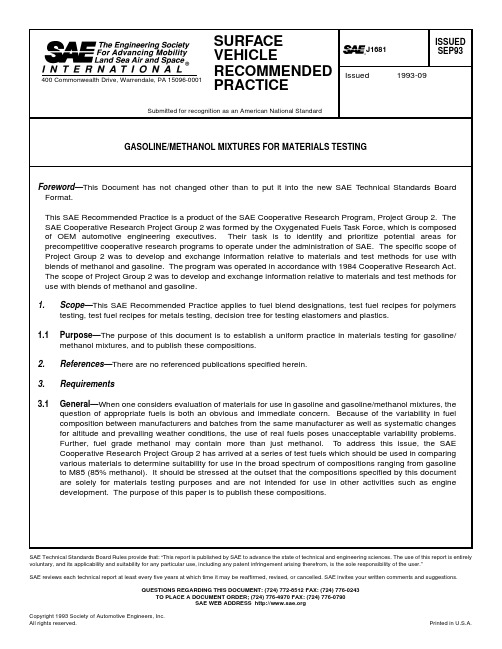
SAE Technical Standards Board Rules provide that: “This report is published by SAE to advance the state of technical and engineering sciences. The use of this report is entirely voluntary, and its applicability and suitability for any particular use, including any patent infringement arising therefrom, is the sole responsibility of the user.”SAE reviews each technical report at least every five years at which time it may be reaffirmed, revised, or cancelled. SAE invites your written comments and suggestions.QUESTIONS REGARDING THIS DOCUMENT: (724) 772-8512 FAX: (724) 776-0243TO PLACE A DOCUMENT ORDER; (724) 776-4970 FAX: (724) 776-0790SAE WEB ADDRESS 3.2Test Fuel Designations/Composition—The basic fuel designations selected are shown in 3.2.1 with specificrecipes which can be readily prepared given in 3.2.2 and 3.2.3. The selected compositions are intended for accelerated materials testing. In order to distinguish the materials test fuels from engine test fuels (e.g., M85), the fuels throughout this document are designated by CM followed by the percentage of methanol. This is shorthand for ASTM Fuel "C" blended with methanol. ASTM Reference Fuel "C" (50% toluene, 50% iso-octane) was chosen as a surrogate for gasoline. The high aromatic content provides a fuel which is aggressive for swelling polymeric materials. Using this fuel will also allow building on the large body of Fuel "C" materials testing data already in existence. Freshly opened reagent grade methanol is used to provide a source of methanol. Because it is anticipated that the methanol actually seen in the field will contain more water than is allowed by the definitions for reagent grade methanol, distilled water is to be added.Various possible contaminants in an actual gasoline can aggravate material deterioration. In addition to the basic set of fuels (3.2.1), aggressive fuels are needed to challenge potential fuel system materials. Chloride ions, formic acid, and water are added to create an aggressive test fuel for metals (3.2.3). Some of the components in gasoline can decompose by a process called auto-oxidation to form aggressive substances which can attack polymers and corrode metals. The formation of free radicals from hydroperoxides is accelerated by the presence of trace metals, such as copper, in the fuel. Therefore, when determining the effect of auto-oxidized fuel on polymers, controlled amounts of copper ions and hydroperoxides are added to the test fuels (3.2.2). For metals, hydroperoxide alone is added to the test fuels (3.2.3).3.2.1F UEL B LEND D ESIGNATIONS3.2.1.1Reference GasolineASTM Reference Fuel "C"50% T oluene50% Iso-Octane3.2.1.2MethanolReagent Grade Methanol3.2.1.3Alcohol/Fuel Blends for Polymers (see 3.2.2 for recipes)CM0 Reference GasolineCM15 15% Methanol/85% Reference GasolineCM30 30% Methanol/70% Reference GasolineCM50 50% Methanol/50% Reference GasolineCM85 85% Methanol/15% Reference Gasoline3.2.1.4Aggressive Fuel for Metals (see 3.2.3 for recipes and definition of Aggressive Methanol)CM15A 15% Aggressive Methanol/85% Reference GasolineCM85A 85% Aggressive Methanol/15% Reference Gasoline3.2.1.5Auto-Oxidized Fuel for Any Material (see 3.2.2 or see 3.2.3 for recipes)CMxx + the suffix P (e.g., CM30P, CM85AP, etc.)3.2.2T EST F UEL R ECIPES FOR P OLYMERS T ESTING3.2.2.1For elastomersTolueneCM0 500 mLCM15 425 mLCM30 350 mLCM50 250 mLCM85 75 mLIso-OctaneCM0 500 mLCM15 425 mLCM30 350 mLCM50 250 mLCM85 75 mLMethanol (Reagent Grade)CM0 0 mLCM15 150 mLCM30 300 mLCM50 500 mLCM85 850 mLDistilled WaterCM0 0 mLCM15 0.15 mLCM30 0.30 mLCM50 0.50 mLCM85 0.85 mL3.2.2.2For plastics, add to each liter of test fuel3 ppm chloride ion 0.005 g NaCl (0.003 g Cl−)30 ppm formic acid 0.050 mL3.2.2.3For auto-oxidized test fuel, add to each liter50 mm of t-Butyl Hydroperoxide - 6.8 mL of 70% t-Butyl hydroperoxide per liter of fuel0.01 mg/L Cu as cuprous sulfate final concentration in fuel3.2.3T EST F UEL R ECIPES FOR M ETALS T ESTING3.2.3.1CM15A 15% Aggressive Methanol/85% Reference Gasoline420 mL toluene420 mL iso-octane150 mL aggressive methanol110 mL distilled water3.2.3.2CM85A 85% Aggressive Methanol/15% Reference Gasoline75 mL toluene75 mL iso-octane850 mL aggressive methanol1To prepare an auto-oxidized test fuel for metals, for each liter of the fuels in 3.2.3.1 and 3.2.3.2 add:10 mm of t-Butyl Hydroperoxide - 1.4 mL of 70% t-Butyl Hydroperoxide3.2.4D ECISION T REE FOR T ESTING E LASTOMERS AND P LASTICSa.For elastomers, use CM0, CM15, CM30, CM50, and CM85.b.For plastics, use CM0, CM15, CM30, CM50, and CM85 with additives shown in 3.2.2.e indicator test to determine worst case fuel (e.g., swelling for elastomers and flex modulus forplastics).d.Do any additional testing in worst case blend. (This would allow comparing each material at its worstagainst other materials at their worst to determine suitability for use in a particular application.)However, if CM0 is the worst case, use both CM0 and CM15 so that effects of methanol may beobserved.3.3Test Fuels for Polymeric Materials—The test fuels for polymeric materials, coupled with a decision treeshown in 3.2.4, minimize testing while leading the engineer to potentially suitable candidate materials. Of course, the choice of final material to be used is dependent on the application as well as the specific design and will likely involve the cooperative efforts of the component engineer, materials engineer, and the suppliers.Flex modulus will be used as a screening test for plastics with the aggressive version of each of the five primary test fuel compositions. The worst case fuel will then be used for subsequent testing. Again, if CM0 is the worst case, use both CM0 and CM15 so that effects of methanol may also be observed.3.4Test Fuels for Metals—The test fuels for metals consist of two aggressive methanol blends and two optionalauto-oxidized aggressive methanol blends (CM15A, CM85A, CM15AP, and CM85AP, respectively). Tests should include immersion in the fuel and exposure to the vapor above the fuel. The test specimens should be examined for corrosion or any obvious chemical attack.3.5Tests for Elastomers—Swelling will be used as a screening test for elastomers with each of the five primarytest fuel compositions. The worst case fuel will then be used for subsequent testing. (This would allow comparing each material at its worst against other materials at their worst to indicate suitability for use in a particular application.) However, if CM0 is the worst case, use both CM0 and CM15 so that effects of methanol may also be observed. It is also prudent to test elastomers with an auto-oxidized test fuel (3.2.2.3).1.Aggressive MethanolReagent Grade Methanol (999 mL)0.1% distilled water(1 mL)3 ppm chloride ion(0.005 g NaCl)60 ppm formic acid(0.050 mL)3.6Other Requirements—The set of test fuels specified in this document are suitable for screening materials foruse with gasoline/methanol fuel mixtures up to 85% methanol. It is recognized that additional test fuels may be needed in the future to deal with special circumstances such as permeability testing.PREPARED BY THE SAE FUEL LINES AND FITTINGS COMMITTEERationale—Not applicable.Relationship of SAE Standard to ISO Standard—Not applicable.Application—This SAE Recommended Practice applies to fuel blend designations, test fuel recipes for polymers testing, test fuel recipes for metals testing, decision tree for testing elastomers and plastics. Reference Section—There are no referenced publications specified herein.Developed by the SAE Fuel Lines and Fittings Committee。
汽车外文文献3
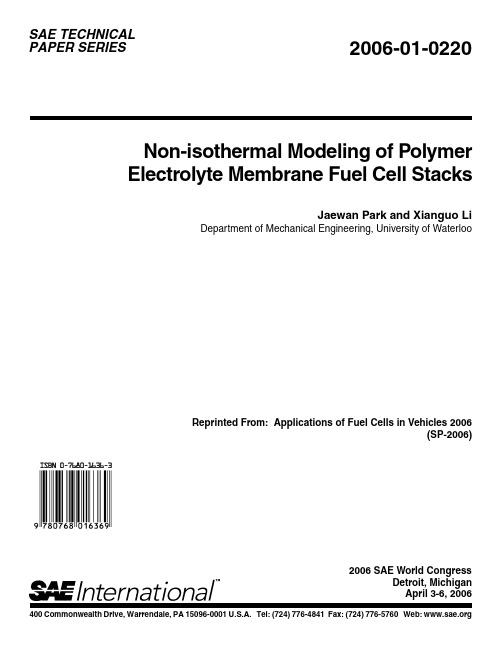
The Engineering Meetings Board has approved this paper for publication. It has successfully completed SAE's peer review process under the supervision of the session organizer. This process requires a minimum of three (3) reviews by industry experts. All rights reserved. No part of this publication may be reproduced, stored in a retrieval system, or transmitted, in any form or by any means, electronic, mechanical, photocopying, recording, or otherwise, without the prior written permi the last few decades, fuel cell has emerged as a leading technology of clean power generation. The polymer electrolyte membrane (PEM) fuel cell is now regarded as a promising alternative of internal combustion engine owing to its competitive power density with high efficiency as well as zero emission [1]. In order to satisfy the power demand of the practical applications, numerous cells should be connected in serial to form a fuel cell stack. The stack performance depends on individual cell output, however, it cannot be estimated from the multiple of single cell operation. The performance degradation may be caused by the unequal supply of fuel and oxidant which results in variation of each cell operating conditions such as pressure, temperature and species concentrations. The stack performance must be analyzed from each cell operation incorporating the function of stack manifold and, in this manner, several single cell models have been extended to stack model. Thirumalai and White developed a performance model of a PEM fuel cell stack [2] by integrating a pipe flow network into the two-dimensional isothermal single cell model of Nguyen and White [3]. It has been shown that the voltage variation is caused by the unequal gas flow to the individual cells in the stack. The generalized steady-state electro-chemical model (GSSEM) of Amphlett et al. [4] has been extended to predict the life of a fuel cell so that the performance
SAE下载论文
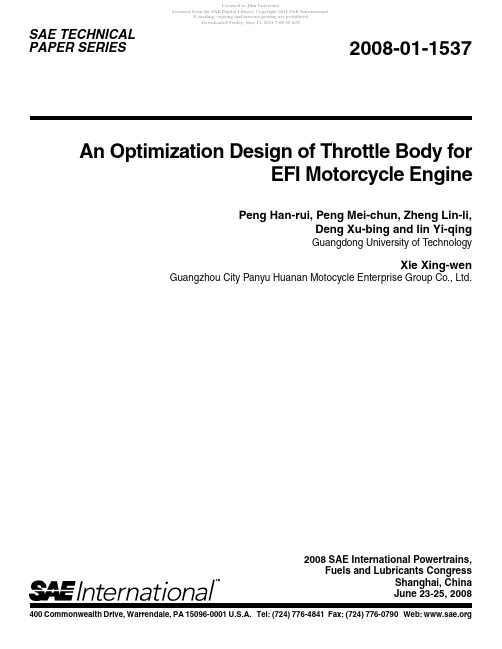
SAE TECHNICALPAPER SERIES2008-01-1537An Optimization Design of Throttle Body forEFI Motorcycle EnginePeng Han-rui, Peng Mei-chun, Zheng Lin-li,Deng Xu-bing and lin Yi-qingGuangdong University of T echnologyXie Xing-wenGuangzhou City Panyu Huanan Motocycle Enterprise Group Co., Ltd.2008 SAE International Powertrains,Fuels and Lubricants CongressShanghai, ChinaJune 23-25, 2008By mandate of the Engineering Meetings Board, this paper has been approved for SAE publication upon completion of a peer review process by a minimum of three (3) industry experts under the supervision of the session organizer.All rights reserved. No part of this publication may be reproduced, stored in a retrieval system, or transmitted, in any form or by any means, electronic, mechanical, photocopying, recording, or otherwise, without the prior written permission of SAE.For permission and licensing requests contact:SAE Permissions400 Commonwealth DriveWarrendale, PA 15096-0001-USAEmail: permissions@Tel: 724-772-4028Fax: 724-776-3036For multiple print copies contact:SAE Customer ServiceTel: 877-606-7323 (inside USA and Canada)Tel: 724-776-4970 (outside USA)Fax: 724-776-0790Email: CustomerService@ISSN 0148-7191Copyright © 2008 SAE InternationalPositions and opinions advanced in this paper are those of the author(s) and not necessarily those of SAE. The author is solely responsible for the content of the paper. A process is available by which discussions will be printed with the paper if it is published in SAE Transactions.Persons wishing to submit papers to be considered for presentation or publication by SAE should send the manuscript or a 300 word abstract of a proposed manuscript to: Secretary, Engineering Meetings Board, SAE. Printed in USA2008-01-1537 An Optimization Design of Throttle Body for EFIMotorcycle Engine Peng Han-rui, Peng Mei-chun and Zheng Lin-li, Deng Xu-bing and lin Yi-qingGuangdong University of TechnologyXie Xing-wenGuangzhou City Panyu Huanan Motocycle Enterprise Group Co., Ltd. Copyright © 2008 SAE InternationalABSTRACTThis paper studied the optimization design of throttle body for electronic control motorcycle engine FY156FMI. It was studied the influence of the inner diameter, configuration of valve and its axis and inner configuration of the pipe of throttle body on the engine performance. The inner diameter of throttle body was designed on the basis of resonance theory. And the prototypes of throttle body designed were manufactured and tested by engine experiment. Results showed that the power of the engine applied with the throttle body, which has been optimization designed with a thinner throttle valve and a taper inlet port, and the size of inner diameter of throttle body increased from 26mm to 28mm and the thickness of the axis decreased, was improved effectively.The optimization throttle body can meet the design target.INTRODUCTIONAir flow has an important influence on the efficiency of engine intake charge, and the engine power and consumption directly. More fresh air could be taken, more fuel could be injected into engine in the same intake condition and same volume of cylinders, then the effective power of engine will be more high with the similar combustion [ 1 ].The intake process is complex and resonant, and it has a connection with the configuration of intake pipe directly. The intake resistance will increase with the diameter reduced and length of the intake pipe increased. And the peak of intake charge coefficient will move to the low velocity [ 2 ]. For this reason, scholars have paid much attention to the configuration of the intake pipe and the dynamic characteristic of the intake flow [3-6] . As a part of the EFI engine intake pipe, the configuration of throttle body has a great influence on the power, torque and fuel consumption of engine.This research was based on a gasoline engine 156FMI for CG125 motorcycle which is a carburetor engine originally. When the engine was changed to EFI engine, it was required to design a throttle body. The target of throttle body design is to ensure that the maximum power of the EFI engine is not lower than the original carburetor engine.INITIAL DESIGN OF THTOTTLE BODYThe throttle body of EFI engine is substitute for the carburetor. Therefore the overall externaldimensions of throttle body are equal to the carburetor. The length of throttle body should be consistent with the carburetor to avoid the modification of vehicle frame and other parts. The design project of throttle body is integrated, namely, the throttle body, throttle positionsensor, manifold pressure sensor and inlet temperature sensor are integrated together .The components structure of integrated throttle body for this research is shown in figure 1. The integrated structure has the advantage of lowcost, good reliability , and easy to install etc..Figure 1 Configuration of the integrated throttle bodyFigure 2 Configuration of the initial design throttle bodyThe inner diameter of carburetor for the original motorcycle engine is ∅26, therefore, inner pipe diameter of throttle body for EFI engine was designed to ∅26 too. The configuration of inlet pipe of throttle body is shown in figure 2.In order to confirm that the throttle body meets the design target of power and fuel consumption. The engine performance was tested on engine test bench. The engine performance was conducted at different speed points, which is properly distributed in speedrange with full throttle open. Usually, more than eight measurement points would be adopted.The Major Specifications of original carburetor engines were shown in table 1. The test equipments included MZDC-22 eddy current dynamometer, MCS-960 fuel consumption meter, which were made in Zhong Cheng Equipment Co., Ltd.Table 1 Major Specifications of prototype engine with carburetor Engine Type FY156FMIFormatsingle cylinder, four stroke, natural aircoolingCylinder Diameter(mm) 56.5Stroke(mm) 49.5 Displacement(mL) 124Compression Ratio 9.0:1Max Power(kW)/ Speed(r/min) 8.1/8500Power Rating(kW)/Speed(r/min) 7.2/8000Max Torque(N.m)/Speed(r/min) 9.35 /8000Min Specific FuelConsumption(g/kW.h)≤365Engine performance comparison was conducted between EFI engine with a ∅26 throttle body and original carburetor engine FY156FMI. The results were shown in figure 3. From the figure 3, it can be seen that the power of the EFI engine with a ∅26 throttle body is lower than that of the original carburetor engine distinctly. So it can’t meet the design target. There are two reasons for the power dropping of EFI engine:•The size of inner diameter of throttle body may be too small.•The configuration of throttle body is unreasonable, There are two stairs inthe inlet port of intake pipe of thethrottle body (as shown in figure 2 ),which causes the air flow resistance.The intake charge of engine isinsufficient and causes the enginepower dropping. Therefore it isimportant to improve the throttle body,Figure 3 Comparison of Full load performances of EFI engine with a ∅26 throttlebody with carburetor engineIMPROVEMENT DESIGN OF THE THROTTLE BODYAfter the basic configuration of throttle body had been set, it is important to improve the design of length, the inner diameter of the throttle body and the internal structure according to the design requirement and the engine technology parameters.The resonance theory was applied to the design of length and the diameter of throttle body. If the frequency of intake system and the cycle of charge are harmonious under specific velocity of engine, it will cause the high wave of pressure in the intake pipe before the intake valve closed, make the pressure in the intake pipe augmented, and increase the engine intake charge. The effect is called resonance theory. The advantage of the resonance intake system is that it can work reliably to increase the intake charge without any moving parts, thereby increase the engine power and the torque with lower cost under specific velocity. The technology parameters of FY156FMI engine show that the velocity at maximum torque is 8000r/min, therefore the research took this velocity to carry on the design of resonant intake pipe to increase the maximum power and the torque of the engine. Because of the length of the throttle body should be consistent with that of carburetor, only the internal pipe diameter design is need.THE DESIGN IMPROVEMENT OF INNNER DIAMETER OF THROTTLE BODYStrictly, the inner diameter of resonant pipe should be designed according to the pipeline friction and the undulation effect. In view of the friction effect, the pressure dropped in the pipeline is proportional to the countdown offourth power of the inner diameter, thus, larger diameter should be adopted. But in other view of pressure undulation effect, smaller diameter should be adopted to increase the pressure undulation amplitude. Just considering the impact of friction, Kastner pointed out thesmallest diameter estimation formula as following:mm n s d d i 51624min107.12)(⎦⎤⎢⎣⎡×=[7] (1) “d” represents the diameter of cylinder, “s” represents the piston stroke, “ n” represents the rotation speed of engine.Put “d =56.5mm, S =49.5mm, n =8000r/min” into the formula (1)to calculate the smallestdiameter. The result is min )(i d =27.5mm, rounds to d i =28mm. And the two staris in the inlet port of the throttle body is removed. The inner configuration of intake pipe designed isshown in figure 4.Figure 4 Throttle body with ∅28 intake pipe OPTIMIZED DESIGN OF THE VALVE AND AXIS OF THROTTLE BODYIn order to reduce the flow resistance, the optimized design of valve and axis of throttle body can refer to the design of choke valve. One option is that the half of axis was cut. The axis is semicircle. Another option is that both sides of axis are beveled. The axis is flat. The two options are contributed to reduce the effect of flow resistance. Also, the thickness of the valve and the size of the axis diameter decreased in optimized design.There is a stair at the inlet port of throttle body in the initial design option. For improving, the side of the pipeline where the valve body connected with the air filter was expanded into a certain degree of taper, to smooth the transition and reduce the air resistance further. Design options of throttle bodies are shown in figure 5.The samples of throttle body design were manufactured and tested by engine experiment to choose the best one. The state of initial design and improvement design options of throttle body are shown in table 2.Table 2 Throttle body design optionsFigure 5 Valve and axis optimization design options of throttle bodyENGINE PERFORMANCE COMPARISON TEST AND DATA ANALYSISFour kinds of throttle bodies, as shown in table 2, were applied to a same FY156FMI EFI engine, and it was tested their effect on performance of engine. The test equipments are the same as above.It is showed the power curves in full load for FY156FMI EFI engine applied with differentthrottle bodies in figure 6. The analysis and results are listed as following:•The engine power improved effectively when the diameter of throttle bodyincreases from 26mm (option 0)to28mm (operation 1, 2, 3), and thegreatest increment is 1.29kW.•The power of EFI engine equipped with only enlarging inner diameter throttlebody(option 1) is lower than that ofcarburetor engine. But the power of EFIengine almost is equal to that ofcarburetor engine, when EFI enginewas equipped with the throttle body witha thin valve and a taper inlet port, andthe size of inner diameter increasedfrom 26mm to 28mm and the thicknessof the axis decreased (option 2 andoption 3),also, the maximal power is alittle larger than the carburetor engine’s.•When the thickness of valve and axis decreased(option 2 and option 3 ), theengine power is increased by 0.2 ~0.3kW. And there are not obviousdifferences of the influence on powerbetween the semicircle axis and the flataxis.Figure 7 shows specific fuel consumption curves of option 2(semicircle axis) and option 3 (flat axis)that EFI engine equipped with throttle body and original carburetor engine. Obviously, after enlarging throttle body inner diameter and decreasing the thickness of the valve and axis, EFI engine specific fuel consumption is distinctly lower than that of the carburetor engine. The specific fuel consumption reduced by 5.8% averagely. Moreover, the specific fuel consumption of the EFI engine equipped with the semicircle axis had only a few fluctuations at the entire test speed scope, especially at the middle and high speed, the specific fuel consumption is almostthe same.Figure 6 Performance of full load of engineFigure 7 Specific fuel consumption comparisonCONCLUSIONSIt can be concluded from the above analysis that the throttle body has a great influence on the EFI engine power and fuel consumption. The paper applied the optimization design, such as increasing inner diameter from 26mm to 28mm, decreasing the thickness of the valve and the axis and smoothing the inside of intake pipe to increase the engine power. The results showed that the power of EFI engine equipped with the optimization design throttle body is almost equal to the carburetor engine. The optimization throttle body can meet the design target. Specially, the fuel consumption of EFI engine equipped with semicircle axis type throttle body is much lower than that of carburetor engine.REFERENCES[1] Yu Guohe, Yan Xiang’an. “Experimental Study on Influence of Inlet Pipe on Dynamic Performance of Single-cylinder Engine”. China Mechanical Engineering, 2006(17) supplement:140-143[2] Zhou Longbao. “Internal-combustion Engine”. Beijing: Machinery Industry Press,1999 [3] Wilson N, Watkins A, Dopson C. “A symmetric valve strategies and their effect on combustion” .SAE Paper 930821.1993[4] Feng Mingzhi, Liu Shuliang. “Experimental Study on In-cylinder Air Flow in Four-valve Spark Ignition Engine”. Journal of Tianjin University. 2000(3):355-359.[5] Pei Pucheng,Liu Shuliang, Fan Yongjian. “An investigation on the tumbling intake ports configuration for multi valve S.I. engine”. Journal of Internal CombustionEngine,1993,47:13-19[6] Zhang huiming, Hong Jiadi. “Studyon the inlet system of improved diesel engine”.Small Internal Combustion Engine,1998(6): 28 - 31. [7]Xiao Min, Zhang Qingsong. “Calculation and Design f or JL368 Q Petrol Engine Intake System”. Journal of China Shipbuilding Institute, 2002,16(1):56-61。
SAEJ1684v001
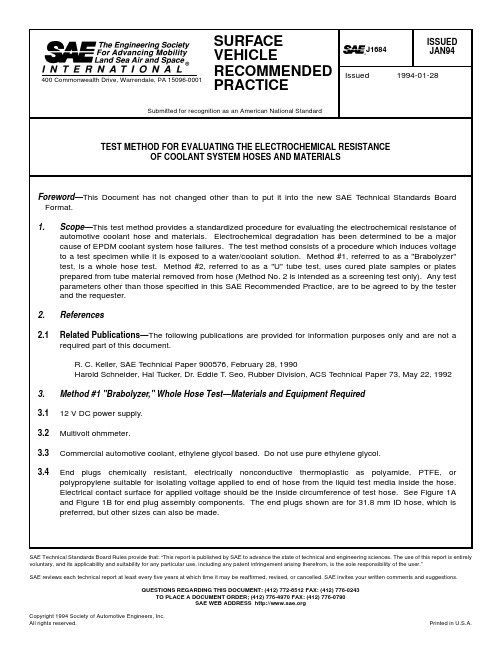
TEST METHOD FOR EVALUATING THE ELECTROCHEMICAL RESISTANCE OF COOLANT SYSTEM HOSES AND MATERIALS
Foreword—This Document has not changed other than to put it into the new SAE Technical Standards Board Format. 1. Scope—This test method provides a standardized procedure for evaluating the electrochemical resistance of automotive coolant hose and materials. Electrochemical degradation has been determined to be a major cause of EPDM coolant system hose failures. The test method consists of a procedure which induces voltage to a test specimen while it is exposed to a water/coolant solution. Method #1, referred to as a "Brabolyzer" test, is a whole hose test. Method #2, referred to as a "U" tube test, uses cured plate samples or plates prepared from tube material removed from hose (Method No. 2 is intended as a screening test only). Any test parameters other than those specified in this SAE Recommended Practice, are to be agreed to by the tester and the requester. References Related Publications—The following publications are provided for information purposes only and are not a required part of this document. R. C. Keller, SAE Technical Paper 900576, February 28, 1990 Harold Schneider, Hal Tucker, Dr. Eddie T. Seo, Rubber Division, ACS Technical Paper 73, May 22, 1992 3. 3.1 3.2 3.3 3.4 Method #1 "Brabolyzer," Whole Hose Test—Materials and Equipment Required 12 V DC power supply. Multivolt ohmmeter. Commercial automotive coolant, ethylene glycol based. Do not use pure ethylene glycol. End plugs chemically resistant, electrically nonconductive thermoplastic as polyamide, PTFE, or polypropylene suitable for isolating voltage applied to end of hose from the liquid test media inside the hose. Electrical contact surface for applied voltage should be the inside circumference of test hose. See Figure 1A and Figure 1B for end plug assembly components. The end plugs shown are for 31.8 mm ID hose, which is preferred, but other sizes can also be made.
SAE paper 外文原文

2003-01-0240
Finite Element Analysis of Static Elastic Characteristics of the Rubber Isolators in Automotive Dynamic Systems
Shangguan Wenbin and Lu Zhen-Hua
Department of Automotive Engineering and State Key Laboratory of Automotive Safety and Energy Conservation, Tsinghua University
Shi Jianjun
Department of Mechanical Engineering, Iowa State University
2003 SAE World Congress Detroit, Michigan March 3-6, 2003
400 Commonwealth Drive, Warrendale, PA 15096-0001 U.S.A. Tel: (724) 776-4841 Fax: (724) 776-5760 Web:
exhaust pipes. The static and dynamic properties of those components have great influence on the performance of ride and handing of a vehicle. So a sophisticated mechanical analysis should be carried out for the components that require high performance and high durability. Based on the classic theory of elasticity, some closed form formulae can be obtained for the static stiffness analysis in radial, axis, torsional and tilting direction of a regular configuration bushing[1,2,16]. But most of those formulae are not suitable for calculating the stiffness of a complex configuration bushing. For a mount of cylinder configuration, its static stiffness can be calculated by the formulae given in references [3,4]. However for complex mounts, no analytical solutions are given. The Finite Element Method (FEM) has been successfully applied to engineering structures when strains are small and materials are linear, and FEM is now also widely used in engineering application associated with large deformation and material nonlinearity. With the development of computer hardware and the model characterizing rubber elastic behaviors, many commercial FEM programs, such as ADINA [8], ABAQUS [6] and MARC [7], are available for Finite Element Analysis (FEA) of rubber productions. The programs were used to carry out the FEA of different rubber components by the authors in references [9] to [13], and most of those applications are limited to static elastic characteristics analysis. There are considerable differences in the FEA of elastomeric components and metal products. (1) Constitutive properties. Within certain range of response, metals are regarded as elastic, linear and isotropic. This implies that normal and shear stress components can be expressed as a linear function of the corresponding strain, and the load-deflection curve for loading and unloading is the same. So only two material constants, such as Young’ s modulus or elastic modulus and Poisson’ s ratio, are needed to characterize the three dimensional response of the metal material and the
SAE J1563-1993 GUIDELINES FOR LABORATORY CYCLIC CORROSION TEST PROCEDURES FOR PAINTED AUTOMOTIVE PAR
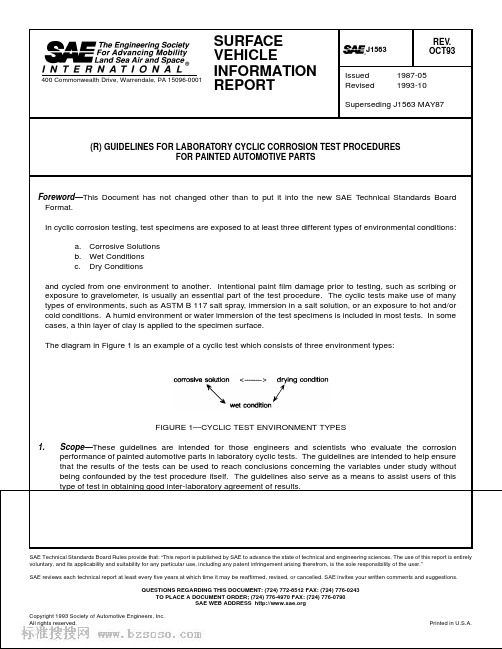
FIGURE 1—CYCLIC TEST ENVIRONMENT TYPES 1. Scope—These guidelines are intended for those engineers and scientists who evaluate the corrosion performance of painted automotive parts in laboratory cyclic tests. The guidelines are intended to help ensure that the results of the tests can be used to reach conclusions concerning the variables under study without being confounded by the test procedure itself. The guidelines also serve as a means to assist users of this type of test in obtaining good inter-laboratory agreement of results.
SAE Technical Standards Board Rules provide that: “This report is published by SAE to advance the state of technical and engineering sciences. The use of this report is entirely voluntary, and its applicability and suitability for any particular use, including any patent infringement arising therefrom, is the sole responsibility of the user.” SAE reviews each technical report at least every five years at which time it may be reaffirmed, revised, or cancelled. SAE invites your written comments and suggestions. QUESTIONS REGARDING THIS DOCUMENT: (724) 772-8512 FAX: (724) 776-0243 TO PLACE A DOCUMENT ORDER; (724) 776-4970 FAX: (724) 776-0790 SAE WEB ADDRESS Copyright 1993 Society of Automotive Engineers, Inc. All rights reserved.
SAE J861-2003

SURFACE VEHICLE STANDARDSAE Technical Standards Board Rules provide that: “This report is published by SAE to advance the state of technical and engineering sciences. The use of this report is entirely voluntary, and its applicability and suitability for any particular use, including any patent infringement arising therefrom, is the sole responsibility of the user.” SAE reviews each technical report at least every five years at which time it may be reaffirmed, revised, or cancelled. SAE invites your written comments and suggestions. Copyright © 2003 SAE InternationalAll rights reserved. No part of this publication may be reproduced, stored in a retrieval system or transmitted, in any form or by any means, electronic, mechanical, photocopying, recording, or otherwise, without the prior written permission of SAE.TO PLACE A DOCUMENT ORDER:Tel: 877-606-7323 (inside USA and Canada)Tel: 724-776-4970 (outside USA) Issued 1963-06 Revised 2003-12Superseding J861 JAN1994Publications2.2 RelatedThe following publications are provided for information purposes only and are not a required part of this document.P UBLICATIONS2.2.1 ASTMAvailable from ASTM, 100 Barr Harbor Drive, West Conshohocken, PA 19428-2959.ASTM D 2645—Standard Tolerances of Yarns Spun on the Cotton or Worsted System2.2.2 T ESTFABRICS I NC.Available from Testfabric, Inc., 415 Delaware Avenue, P.O. Box 26, W est Pittston, PA 18643, 570-603-0432, Fax: 570-603-0433.2.2.3 SDLA TLAS, (T EXTILE I NNOVATORS C ORPORATION)Available from SDL Atlas, 1813A Associates Lane, Charlotte, NC, 28217, 704-329-09113. Materials and Equipment3.1 AATCC CrockmeterA device which has a 16 mm ± 1 mm diameter rubbing peg, reciprocating in a straight line rubbing motion, to and fro along a 104 mm ± 3 mm track, with a downward force of 9 N ± 0.9 N.3.2 Crockmeter Test ClothPlain weave 80x84 greige (32 ends/cm ± 3 ends/cm and 33 picks/cm ± 3 picks/cm.) 15 Tex ± 4% yarn size, 5.9 turns/cm, Z ± 10% twist, combed cotton, desized, bleached, without finish, and cut into nominal 50 X 50 mm squares. Cloth should have a whiteness rating of 80 or above per AATCC Method 110. 3.3 AATCC Textile Blotting Paper3.4 AATCC Chromatic Transfer Scale3.5 AATCC Gray Scale to Staining3.6 Distilled WaterpH of 6.5 to 7.53.7 Transparent Adhesive Tape(optional)4. TestSpecimen4.1 A minimum of two specimens are required, one for a dry crock test and one for a wet crock test.Specimen shall be cut 51 X 127 mm with the long dimension 45° nominal, to the machine or cross machine (warp and fill) direction. Additional specimens may be used when higher precision isneeded.4.2 In addition, materials with directional patterns (color, texture, nap) should be cut in machine, cross-machine and 45°, two specimens (one dry and one wet) in each direction.4.3 Specimen should be as flat as possible.5. Conditioning5.1 Prior to testing, condition the test specimens and the crock squares for at least 4 h in anatmosphere of 21 °C ± 2 °C and 50%R.H. ± 5% R.H. by laying each test specimen or crock square separately on a screen or perforated shelf of a conditioning rack.5.2 Alternate parameters for conditioning may be specified such as 21 °C ± 2 °C and 65% R.H. ±5% R.H. or as agreed upon by contractual parties. Results may differ from items conditioned in parameters of 5.1 above.6. Procedures6.1 Dry Crock Test6.1.1 Place the test specimen with the long dimension in the direction of the rubbing on the base of theCrockmeter and so that it is flat and uniformly in contact with the abrasive cloth mount.6.1.2 Fasten a dry crock cloth over the end of the peg with the weave parallel to the direction of therubbing. Use the spiral wire clip to hold the test square in place.6.1.3 Lower the peg into contact with the test specimen and turn the crank at a rate of one turn persecond so that the peg moves 10 cycles, (10 times to and 10 times fro) on the test specimen. Ifthe crockmeter is motorized, set the counter for 10 cycles and turn on the machine making sure that the peg is positioned to give a complete 10 cycles. Contracting parties may specify othertest duration’s.6.1.4 Remove the crock square from the peg and condition per Section 5. In the case of napped,brushed, sanded or flocked material with loose fibers that might interfere with the rating, remove extraneous material by pressing lightly on the crock square with the sticky side of transparentadhesive tape before evaluating.6.2 Wet Crock Test6.2.1 Establish a technique for preparing wet crock cloth squares by weighing a preconditioned square,then thoroughly wet out in distilled water. Prepare one square at a time.6.2.2 Bring wet pickup to 65% ± 5% (or as agreed upon by contractual agreement) by squeezing wettesting square between blotting paper.6.2.3 Using the prepared wet testing square, continue as directed in 6.1.1 through 6.1.3.6.2.4 Air dry the crock cloth square, then condition per Section 5 before evaluating. In the case ofnapped, brushed, sanded or flocked material with loose fibers that might interfere with rating, remove the extraneous material by pressing lightly on the crock square with the sticky side of transparent adhesive tape before evaluating.7. Evaluation7.1 Back each test square with three layers of white crocking cloth during the evaluating process. 7.2 Assess visually or instrumentally the color transfer from the specimen to the crock square.7.2.1 For visually assessing the color transfer, use suitable illumination (SAE J361, Section 4) andeither AATCC Gray Scale to Staining or AATCC Chromatic Transfer Scale.7.2.2 For instrumentally assessing the color transfer, SAE J1767 should be used as a guide. Theinstrument port size must be less than 15 mm.7.2.3 Halo effect color change shall not be measured or reported.8. ReportShould include the following:8.1 Test description, whether dry and/or wet crocking test.8.2 Evaluation results per Section 7.8.3 Number of cycles.8.4 Conditioning of specimen and crock cloth.8.5 Material description.8.6 Equipment used - Instrument, make and model, port size, and viewing geometry if instrumentalcolor difference is used for rating.9. NotesVerification of the operation of the test and the apparatus should be made routinely and the results kept in a log. Use an in-house or established crocking calibration specimen and conduct three dry tests.Indicia9.1 MarginalThe change bar (|) located in the left margin is for the convenience of the user in locating areas where technical revisions have been made to the previous issue of the report. An (R) symbol to the left of the document title indicates a complete revision of the report.PREPARED BY THE SAE TEXTILES AND FLEXIBLE PLASTICS COMMITTEERationaleTotal rewrite of previously issued document, reaffirmed January, 1994.Relationship of SAE Standard to ISO StandardNot applicable.ApplicationThis test method can be used to determine the resistance to crocking (color rub-off) of organic trim materials such as fabrics, vinyl coated fabrics, leather, coated fiberboard and carpet.Reference SectionSAE J361—Visual Color Match to Master Specimen for FabricsSAE J1767—Instrumental Color Difference Measurement for Colorfastness of Automotive Interior Trim MaterialsAATCC Method 8—Colorfastness to CrockingAATCC Method 110—Whiteness of TextilesAATCC Chromatic Transfer Scale, AATCC Gray Scale to Staining, and Blotting PaperASTM D 2645—Standard Tolerances of Yarns Spun on the Cotton or Worsted SystemDeveloped by the SAE Textiles and Flexible Plastics Committee。
SAE燃料电池汽车加氢相关标准简析
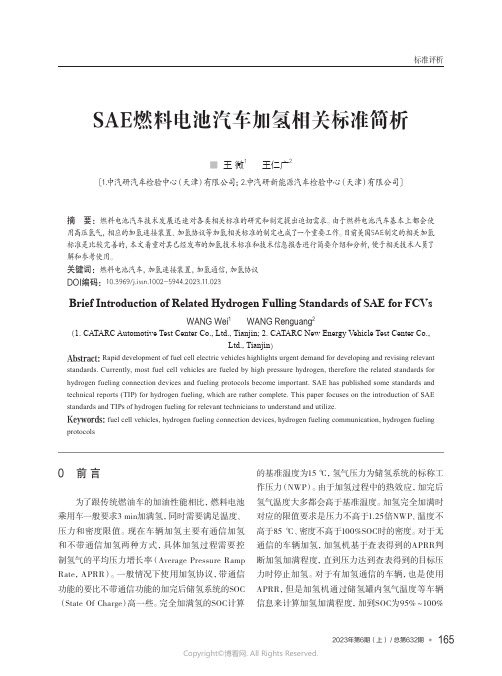
标准评析SAE燃料电池汽车加氢相关标准简析■ 王 微1 王仁广2〔1.中汽研汽车检验中心(天津)有限公司;2.中汽研新能源汽车检验中心(天津)有限公司〕摘 要:燃料电池汽车技术发展迅速对各类相关标准的研究和制定提出迫切需求。
由于燃料电池汽车基本上都会使用高压氢气,相应的加氢连接装置、加氢协议等加氢相关标准的制定也成了一个重要工作。
目前美国SAE制定的相关加氢标准是比较完善的,本文着重对其已经发布的加氢技术标准和技术信息报告进行简要介绍和分析,便于相关技术人员了解和参考使用。
关键词:燃料电池汽车,加氢连接装置,加氢通信,加氢协议DOI编码:10.3969/j.issn.1002-5944.2023.11.023Brief Introduction of Related Hydrogen Fulling Standards of SAE for FCVsWANG Wei1 WANG Renguang2(1. CATARC Automotive Test Center Co., Ltd., Tianjin; 2. CATARC New Energy Vehicle Test Center Co.,Ltd., Tianjin)Abstract: Rapid development of fuel cell electric vehicles highlights urgent demand for developing and revising relevant standards. Currently, most fuel cell vehicles are fueled by high pressure hydrogen, therefore the related standards for hydrogen fueling connection devices and fueling protocols become important. SAE has published some standards and technical reports (TIP) for hydrogen fueling, which are rather complete. This paper focuses on the introduction of SAE standards and TIPs of hydrogen fueling for relevant technicians to understand and utilize.Keywords: fuel cell vehicles, hydrogen fueling connection devices, hydrogen fueling communication, hydrogen fueling protocols0 前 言为了跟传统燃油车的加油性能相比,燃料电池乘用车一般要求3 min加满氢,同时需要满足温度、压力和密度限值。
SAE_paper_2006-01-0745_Wattard

2006-01-0745 Development of a 430cc Constant Power Engine for FSAECompetitionWilliam Attard and Harry C. WatsonUniversity of Melbourne Copyright © 2005 SAE InternationalABSTRACTThis paper describes the design and development of an engine with constant power for SAE’s student Formula race-car competition, allowing the avoidance of gear shifting for much of the Autocross event. To achieve constant power for over 50% of the speed range, turbocharging was adopted with a boost pressure ratio of 2.8 at mid-range speeds and applied to an engine capacity of 430 cc. This engine was specifically designed and configured for the purpose, being a twin cylinder in-line arrangement with double overhead camshafts. Most of the engine components were specially cast or machined from billets. The capacity was selected to minimise frictional losses and thus increase delivered power along with dry sump lubrication and a three speed gear box. The engine manifolds and plenums were designed using a CAE application and proved to be well suited to the task resulting in excellent agreement between predicted and actual performance. One of the major challenges of the experimental development was overcoming the turbocharger oil consumption under throttled operation at part load conditions and at full power when the FSAE restrictor is choked. For the 2004 Australian competition the engine was run with slightly reduced mid speed power to avoid excessive use of the traction control system and was very competitive finishing first in the fuel economy event. INTRODUCTIONFormula SAE has proven itself to be a problem based learning exercise that employers regard as being equivalent to up to eighteen months of work-place training. It can also be an opportunity for under-graduate students to learn research skills in preparation for a career in research and teaching.This paper focuses on the design, research and development of a purpose designed engine for FSAE and demonstrates that the challenge of looking for new ways to meet the FSAE rules and requirements can be more than a training experience. The Formula Rules limit the engine capacity and air consumption by means of a restriction in the intake system. Specifically the engine should:Be a four-stroke piston engineBe limited to a maximum displacement of 610ccUse gasoline fuel limited to 98 RONHave all intake air passing through a 20mmrestrictionHave the throttle located upstream of the restrictorAllow superchargingThe major event in FSAE is the Autocross, which has an average speed of around 55 km/h which typically involves a speed range varying from 30 to 90 km/h. In vehicle acceleration over this speed range, typically two or more gear up shifts (and downshifts) will be needed. It is noted that automatic gear shifting in motor sport major-competition typically takes about 30 milliseconds, so in one lap up to 0.5 seconds may be ‘lost’ to gear shifting. Even if gearshifts are made clutchless, with the drive train absorbing the shocks, the torque pulsations unsettle the vehicle stability particularly in turns. It follows that an engine with constant power over the speed range has a potential advantage in the autocross event by eliminating gear shifting and running in only one gear. However in the acceleration event, a gear change may be required depending on traction limitations.Thus the objectives of a turbocharged engine design compared with a 4-cylinder 600 cc reference engine were set as follows:The engine should be capable of operating at constant maximum airflow (choked) over at least half of the speed range to maximumPower increased by 5-10% through reduced FMEP (friction) through using a smaller than 610cc capacity 25% reduction in mass50% reduction in physical size25% reduction in the height of the COGDesign to suit a FSAE styled race-car with regardsto packaging Maximum throttle response making the engine astractable as possible The design drew on the team’s previous experience in producing small turbocharged engines for a larger engine task, except that FSAE engines are significantly smaller and there was much to learn [1,2,3]. In practice this concept involved a design from scratch approach since no suitable production engine could be adapted. In reality, production items from a range of different engines were sourced as parts together with the design and manufacture of many major and minor components.THEORETICAL ANALYSISCHOICE OF ENGINE CAPACITY AND CONFIGURATIONThe engine capacity was selected with the aid of Figure 1, which shows the volumetric efficiency (as achieved by increasing boost pressure as the engine size reduces to achieve the same power output as a 600 cc engine. A validation point for the simple model simulation is shown with an experimental result from the team’s previous Suzuki GSX-R600 engine at the speed at which the restrictor limits power. On the basis that achieving boost pressures ratios of 3.2 (or VE of 250% assuming losses) is the expected limit from the relatively small, and therefore less efficient turbocharger, an engine size of between 400 and 450 cc was the design brief with an operating speed range of 6000-10000 rev/min.delivery pressure) needed to achieve sonic flow in the restrictor.An increase in the number of cylinders reduces the flow velocity fluctuations experienced by the exhaust turbine. However, with frictional losses increasing with an increase in the number of cylinders and resultant piston rubbing area, a compromise between one and four was needed, yielding a two cylinder configuration.MANIFOLD DESIGNSThe design of the plenums in between the compressor, engine and turbine were an important consideration to offset the flow pulses especially as an uneven firing order was selected for engine balance reasons. Each of the plenums adopted Watson’s KEC rolling flow design where the kinetic energy of the flow is conserved in a vortex about the axis of the plenum. The design can be seen pictorially in the CAD image of Figure 2. To determine the size of the plenums, manifold design and valve timing events, an extensive series of simulations were undertaken using Ricardo’s WAVE ®. The predicted results with relatively large plenums are shown in Figure 3.Figure 2: CAD image (upper) of the ‘WATTARD’ turbocharged engine showing the turbocharger location and the manifold and plenum designs. Lower picture shows the turbocharger location and exhaust plenum behind the dry sump oil tank.Figure 3: Simulation results for the WATTARD TC engine andthe team’s previous Suzuki GSX-R600 engine with GSX-R600experimental results used for model validation. ENGINE DESIGN AND SPECIFICATIONThe specification of the engine can be seen in Table 1.Table 1: Final specification for the 2004 TC ‘WATTARD’ engine for FSAE-A competition.Ricardo WAVE Simulations10203040506070The initial stroke of the engine was dictated by theselection of a two-cylinder Kawasaki ER500 crankshaftas an interim measure prior to machining a new item. The gears and change mechanism in the crankcasewere significantly modified with new gear sets to give a wide ratio 3 speed box, compatible with the constantpower concept of the engine.PISTON AND CYLINDERThe twin cylinder barrel was machined from solid featuring a gasketless interface used with success tocontain the high combustion pressures and temperatures associated with turbocharging, with the arrangement as depicted in Figure 4. The oil jet cooledcustom forged pistons received considerable development. The third and final design featured areduced compression ratio of 10.2:1 with an increase inthe number of rings from two to three to reduce blow-by and improve heat rejection at the high boost pressures employed.Figure 4: Section view highlighting the novel Barrel liner/cylinder head interface sealing arrangement.The specially designed and machined connecting rods are shown in Figure 5 together with analysis in Figure 6. At the same mass, the Ι section rod had lower compressive stresses at peak load, just after peak cylinder pressure and thus was adopted.Figure 5: The special requirements and operating conditions of this engine facilitated the design, manufacture and development of many special components including the connecting rod, barrel and piston assemblies depicted above.400050006000700080009000100001100012000Engine Speed (rev/min)Power (kW)BRAND UniMelb “WATTARD” ENGINE TYPE Parallel twin 4 stroke Liquid cooledFIRING ORDER Unequal (0°, 180°CA) CAPACITY 434 ccBORE & STROKE 69 x 58mmBORE/STROKE RATIO 1:1.2 COMPRESSION RATIO10.2:1 VALVE ACTUATION 8-valve DOHCBucket shim adjustmentVALVE TIMING IVO 24° BTDCIVC 72° ABDC EVO 57° BBDCEVC 9° ATDCLUBRICATION Dry SumpOPERATING RANGE 6000 - 10500 rev/min CONROD LENGTH116.5mmINLET20mm restrictor- Dall VenturiWatson KEC manifold with EFI 4.5L plenum volume350mm primary intake length EXHAUSTWatson KEC manifold2.5L plenum volume ENGINE MANAGEMENT Motec M4 EMS TURBOCHARGER Modified Garrett GT12 CLUTCH Multi wet plate (8)TRANSMISSION 3 forward gears50-75 µmCylinder Head Interference Water Cooling Gudgen Pin Connecting RodCONNECTING ROD ALALYSIS1) H - Beam 2) Ι - BeamFigure 6: Evaluation of H and Ι beam connecting rods favoring the adopted latter design.CLUTCH ASSEMBLYThe increase in torque levels necessitated an improved clutch design. The following changes to the wet clutch were made through a series of development steps: The number of friction plates was increased to 8. The clutch plate material was changed to Kevlar. The clutch pressure plate spring stiffness wasincreased by 60%. The clutch basket was reinforced with acircumferential ring to resist the three-fold increase in torque experienced by the clutch.A central oil feed was added to the clutch housingtogether with drillings into the clutch hub to increase oil cooling. The clutch housing with reinforced basket as shownpictorially in Figure 7.Figure 7: Reinforced clutch housing required to transmit theincreased torque of the turbocharged engine.TURBOCHARGERTENSION CONSTRAINTSA well-recognised problem in turbocharging FSAE engines arises from the rules, which dictate that the throttle must be on the suction side of the compressor. Almost all passenger car applications are throttleless (compression ignition engine application) or are upstream of the throttle. The consequence of the upstream throttle is that oil from the compressor side of the journal bearing is sucked into the inlet manifold when the engine is throttled. Not only is this an imposition on the amount of oil that needs to be carried, but the oil causes major combustion problems ranging from plug fouling to pre-ignition and/or increased propensity forknock to occur.BENDING CONSTRAINTSEven though the original metal seal was replaced by a proprietary carbon seal, the vacuum at idle and under restrictor limited choked operating conditions, was such that several litres per hour of oil were consumed. This problem was finally overcome by a novel redesign of the compressor-side seal that normally consists of a split ring seal on the shaft. The seal housing was modified to contain two seals in series with a special venting arrangement between both seals. Because of the turbocharger’s very small size, precision machining of the housing and venting feature were required.The boost pressure was regulated using the waste gate to pressures determined by the information received by the Motec engine management unit. The boost pressure was regulated for the competition to less than the engine’s maximum torque capability, as in second gear wheel spin could be induced over almost all of the speed range. This will be shown in the performance graphs which follow.ENGINE PERFORMANCEThe design and development of the engine took place over a two-year period. For the 2003 Australian competition, the engine ran in NA form and suffered knock/cooling problems, which were eventually overcome.Figure 8: Performance of the WATTARD TC engine at sonicflow conditions above 6000 rev/min compared with the NA version of the engine that ran in the 2003 FSAE-A competition and the team’s previous four-cylinder engine.The turbocharged engine development commenced with a separately driven supercharged version to establish parametric constraints, followed by an extensive period of trial and error solutions to overcome the turbocharger on-throttle oil consumption problem mentioned above. Whilst there is much to be learnt from these experiences, the reader is likely interested in the final version of the engine as it was readied for the 2004 competition.Figure 8 demonstrates the engine’s ability to deliver almost constant power over the 6000 to 10000 rev/min speed range. At 6000 rev/min with a boost pressure ofjust under two atmospheres, the engine’s peak torque is2.6 times that of the naturally aspirated version. From this speed upward the torque falls as the power is limited to about 60 kW or 80 HP. The BMEP diagram represented in Figure 9 compares the specific work performance of a range of recently tested engines. The relatively high BMEP of the NA version of the engine was obtained from a combination of optimised port design, cam timing and low engine friction to produce higher BMEP values at lower speeds when compared to engines normally used for this formula. This was known to be important to obtain the best possible torque before the turbocharger produces waste-gate limited boost. [2] When the car for the 2004 competition was completed and fitted with the 15kg lighter engine, it was apparent that the torque deliverable at low speeds was excessive as can be seen in Figure 10. This shows the torque from the engine with boost controlled via the MOTEC EMS compared with the NA version of the engine and the teams previous four-cylinder engine. Some wheel spin limited torque requirements for intermediate gear operation are plotted.Figure 9: BMEP of the WATTARD TC engine at sonic flow conditions above 6000 rev/min compared with the NA version of the engine and the team’s previous engines.010203040506070809010020003000400050006000700080009000100001100012000Engine Speed (rev/min)Torque (Nm)Power (kW)3000400050006000700080009000100001100012000Engine Speed (rev/min)Figure 10: Performance of the WATTARD TC engine with electronically controlled waste gate operation limiting output for the 2004 FSAE-A competition.COMPARISON OF RESULTS WITH SIMULATIONFigure 11 compares the simulated results displayed in Figure 3 with the performance data of Figure 8. The agreement between the two is excellent except at 10000 rev/min when the actual power tends to fall faster than simulated. This may be caused by mechanical problems related to component flexure and thus increased frictional losses. The importance of the simulation in setting the engine configuration is given with the following example.The size and shape of the exhaust plenum as optimised in the simulation was extremely important due to scavenging and unequal pulse effects attributed to the uneven firing order of this engine. As a result of thermal cracking, the manifold wall thickness of the plenum had to be increased and the material changed from 316 stainless steel to low carbon mild steel. Constructing the three exhaust plenums was very time consuming and thus the plenum was disregarded during development in favour of a simple two into one manifold to save both time and weight. With this new manifold, under all operating conditions, engine torque was down by asmuch as 20% at some speeds with severe knock problems at previously determined MBT values.010203040506070809020003000400050006000700080009000100001100012000Engine Speed (rev/min)Torque (Nm)020406080100120140160180200Power (kW)CFD vs EXPERIMENTAL10203040506070400050006000700080009000100001100012000Engine Speed (rev/min)Power (kW)Figure 11: Comparison of developed and simulated engine performance for both the WATTARD and GSX-R600 engines.CONCLUSIONSA major undertaking for SAE’s student Formula racecar competition – the development of an engine with constant power has been described. The principal object was to run most of the competition in one gear allowing the avoidance of gear shifting for much of the autocross event. A purpose designed and built two-cylinder engine with a capacity of 430 cc and a Garrett GT12 turbocharger was adopted.To obtain a constant power of around 60 kW, a boost pressure ratio of 2.8 was adopted at mid-range engine speeds. This was actually larger than required due to the light car into which it was installed and the reduction in engine mass contributing to the overall mass reduction. Thus for the 2004 competition, the engine had its peak torque de-rated from 90 to 70 Nm.The engine manifolds and plenums were designed using a CAE application and proved to be well suited to the task resulting in excellent agreement between predicted and actual performance. One of the major challenges of the experimental development was overcoming the turbocharger oil consumption under throttled operation at full power when the FSAE restrictor is choked and under part load conditions. This was achieved with a two stage sealing ring with a special venting arrangement between both rings.In general this was a fun project that most FSAE developers might aspire to. Few would have the dedication and experience to succeed. For the 2004 Australian competition, the engine and car were very competitive finishing first in the fuel economy event. This version of the engine and trophy are seen in Figure 12. More development time was needed for the overall car to achieve its best on track performance.Figure 12: The University of Melbourne “WATTARD” engine together with the fuel economy trophy from FSAE-A 2004.ACKNOWLEDGMENTSWhen the second author brought the US formula to Australia he had hoped that 10 Universities might eventually participate in this competition. It was hoped that the participating teams would enjoy much of the practical training that the authors brought to this project from their involvement in different aspects of motor racing. We are pleased to report in 2005 that every University in Australia with a relevant department is participating in this competition. A great credit to its US originators and SAE support from the President, officers, and volunteers of SAE-A.The authors are greatly indebted to the following sponsors:ACL Bearing Company - Tas, Australia APEP Pistons - Vic, Australia Argo Engineering - NSW, Australia Bishop Innovation - NSW, Australia Bohler Uddeholm - Vic, Australia CadCore Pty Ltd -Vic, Australia Concentric Asia Pacific - SA, Australia Cosway Motorcycles - Vic, Australia Davies Craig - Vic, Australia Electromold - Vic, Australia Farley Laserlab - Vic, Australia Garrett Honeywell - NSW, Australia Kawasaki Australia -NSW, Australia Magnesium Technologies -Vic, Australia MAME Centre for Manufacturing - Vic, Australia M&W Ignitions - NSW, Australia Penrite Lubricants - Vic, Australia QMI Solutions - Qld, Australia Ricardo -Sussex, UK Robert Bosch - Vic, Australia Siemans VDO -Vic, Australia University of Melbourne - Vic, Australia Wade Camshafts -Vic, Australia Whitehorse Industries -Vic, AustraliaNOMENCLATUREBMEP brake mean effective pressure CA crank angle COG center of gravityDOHC double over head camshafts EFI electronic fuel injectionEMS engine management system EVC exhaust valve closed EVO exhaust valve openFMEP friction mean effective pressure IVC inlet valve closed IVO inlet vale openKEC kinetic energy conservationMBT minimum spark advance for best torque NA naturally aspirated RON research octane number TC turbo charged VE volumetric efficiencyREFERENCES1. WATSON. H.C., MILKINS, E.E., ROBERTS, K. andBRYCE, W. 'Turbocharging for fuel efficiency, SAE paper 830014. (1983) 2. ROSENKRANZ, H.G., WATSON, H.C., BRYCE, W.and LEWIS, A. 'Driveability fuel consumption and emissions of 1.3 litre turbocharged spark ignition engine developed as a replacement for a 2 litre normally aspirated engine.' Proc. I.Mech.E., C118/86:139-150 pp (1986) 3. ROSENKRANZ, H.G. and WATSON, H.C.Performance Comparison of Ceramic and Conventional Turbines in the Turbocharger of a 1.3L S.I. Engine. Mech.Eng. Dept., Univ. of Melbourne. Report T73/85 (1985)CONTACTProf. Harry Watson, Head Thermofluids Group, Department of Mechanical and Manufacturing Engineering, University of Melbourne, Victoria, Australia 3010. harrycw@.au。
回流式无级自动变速传动系统 毕业设计(论文)1.docx
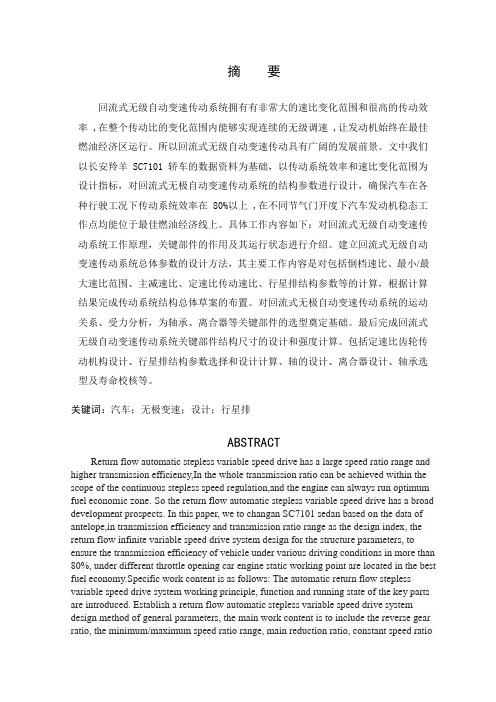
摘要回流式无级自动变速传动系统拥有有非常大的速比变化范围和很高的传动效率 ,在整个传动比的变化范围内能够实现连续的无级调速 ,让发动机始终在最佳燃油经济区运行。
所以回流式无级自动变速传动具有广阔的发展前景。
文中我们以长安羚羊 SC7101 轿车的数据资料为基础,以传动系统效率和速比变化范围为设计指标,对回流式无极自动变速传动系统的结构参数进行设计,确保汽车在各种行驶工况下传动系统效率在 80%以上 ,在不同节气门开度下汽车发动机稳态工作点均能位于最佳燃油经济线上。
具体工作内容如下:对回流式无级自动变速传动系统工作原理,关键部件的作用及其运行状态进行介绍。
建立回流式无级自动变速传动系统总体参数的设计方法,其主要工作内容是对包括倒档速比、最小/最大速比范围、主减速比、定速比传动速比、行星排结构参数等的计算,根据计算结果完成传动系统结构总体草案的布置。
对回流式无极自动变速传动系统的运动关系、受力分析,为轴承、离合器等关键部件的选型奠定基础。
最后完成回流式无级自动变速传动系统关键部件结构尺寸的设计和强度计算。
包括定速比齿轮传动机构设计、行星排结构参数选择和设计计算、轴的设计、离合器设计、轴承选型及寿命校核等。
关键词:汽车;无极变速;设计;行星排ABSTRACTReturn flow automatic stepless variable speed drive has a large speed ratio range and higher transmission efficiency,In the whole transmission ratio can be achieved within the scope of the continuous stepless speed regulation,and the engine can always run optimum fuel economic zone.So the return flow automatic stepless variable speed drive has a broad development prospects. In this paper, we to changan SC7101 sedan based on the data of antelope,in transmission efficiency and transmission ratio range as the design index, the return flow infinite variable speed drive system design for the structure parameters, to ensure the transmission efficiency of vehicle under various driving conditions in more than 80%, under different throttle opening car engine static working point are located in the best fuel economy.Specific work content is as follows: The automatic return flow stepless variable speed drive system working principle, function and running state of the key parts are introduced. Establish a return flow automatic stepless variable speed drive system design method of general parameters, the main work content is to include the reverse gear ratio, the minimum/maximum speed ratio range, main reduction ratio, constant speed ratiotransmission ratio, a key structure parameters calculation, etc. According to the calculation results complete drive system structure of overall draft layout. The return flow of the infinite variable speed drive system motion relations, force analysis, selection of key parts such as bearings, clutch lay the foundation. Finally complete the return flow automatic stepless variable speed drive system design and strength calculation of key components structure size. including constant speed ratio, planetary gear transmission mechanism design structure parameter selection and design calculation, the design of the shaft, clutch design, selection and bearing life and checking.Key words:car;CVT;design; Planet row目录目录.................................................................... 摘要.................................................................... Abstract...............................................................1. 绪论.................................................................1.1 课题的意义......................................................1.2 无极自动变速器的发展............................................1.3 回流式无极自动变速传动系统的结构组成............................1.3.1 金属带式CVT概述...............................................1.3.2 回流式无极自动变速传动系统的结构组成...........................1.3.3 回流式无极自动变速传动系统的特点...............................1.3.4 回流式无极自动变速传动的发展及国内外现状......................1.4 论文的主要研究工作内容..........................................2. 回流式无极变速传动的参数设计.........................................2.1 回流式无极变速传动系统的工作原理................................2.1.1 回流式无极变速器的工作原理....................................2.2 传动系统参数设计................................................2.2.1 传动系统最大速比的计算........................................2.2.2 传动系统最小速比的计算........................................2.2.3 传动系统倒档速比的计算........................................2.3 长安羚羊SC7101轿车原始数据......................................2.4 回流式无极自动变速传动系统的结构参数设计.........................2.4.1带值计算结果……...............................................2.4.2 定速比齿轮参数的确定..........................................2.4.3 行星齿轮参数的确定............................................2.4.4 结构参数的圆整……............................................3. 金属带无极变速装置的力学分析.........................................3.1 金属带主、从动轮的受力分析.......................................3.1.1 CVT传动的运动分析..............................................3.1.2 金属带的轴向力计算............................................3.2 CVT主、从动轴上的轴向力分析......................................4. 回流式无极变速传动系统的关键零部件设计...............................4.1 离合器、制动器的设计............................................4.1.1 理论转矩的计算................................................4.1.2 湿式离合器的设计..............................................4.1.3 制动器的设计..................................................4.1.4 单向离合器的设计..............................................4.2 齿轮的设计......................................................4.2.1 定速比齿轮的设计..............................................4.2.2 行星排的设计..................................................4.3 轴的设计........................................................4.3.1 轴I的设计....................................................4.3.2 轴Ⅱ的设计....................................................1绪论1.1 课题的意义金属带式无级自动变速传动是20世纪80年代末期出现的一种新型汽车传动方式, 它可以让发动机在最佳燃油经济线上运行,使汽车拥有一个没有“漏洞”的牵引特性,来达到节省燃料、减少废气排放的目的;同时, 加速的时候不需切断动力,使轿车不仅乘坐舒适且超车加速性能好,可以减轻驾驶员的驾驶疲劳,提高行车的安全性,因而一直倍受人们的关注[1]。
SAE J2334-2016

SAE WEB ADDRESS:
SAE INTERNATIONAL
J2334™ APR2016
1 of 9
_________________________________________________________________________________________________
2.1.2 ASTM Publications—Available from ASTM, 100 Barr Harbor Drive, West Conshohocken, PA 19428-2959.
ASTM D 1193—Specification for Reagent Water ASTM D 1654—Method for Evaluation of Painted or Coated Specimens Subjected to Corrosive
SAE reviews each technical report at least every five years at which time it may be revised, reaffirmed, stabilized, or cancelled. SAE invites your written comments and suggestions.
SURFACE VEHICLE STANDARD
J
APR2016
Issued Revised Stabilized
1998-06 2003-12 2016-04
Superseding J2334 DEC2003
Laboratory Cyclic Corrosion Test
RATIONALE
GM 9540P— Accelerated Corrosion Test