p450
细胞色素p450还原酶和细胞色素p450
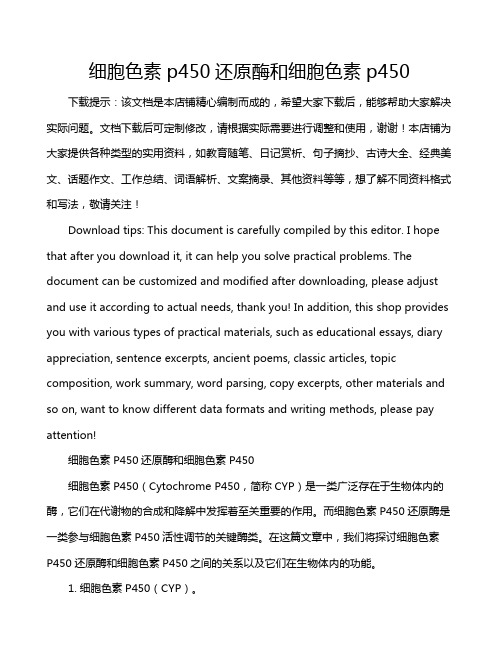
细胞色素p450还原酶和细胞色素p450 下载提示:该文档是本店铺精心编制而成的,希望大家下载后,能够帮助大家解决实际问题。
文档下载后可定制修改,请根据实际需要进行调整和使用,谢谢!本店铺为大家提供各种类型的实用资料,如教育随笔、日记赏析、句子摘抄、古诗大全、经典美文、话题作文、工作总结、词语解析、文案摘录、其他资料等等,想了解不同资料格式和写法,敬请关注!Download tips: This document is carefully compiled by this editor. I hope that after you download it, it can help you solve practical problems. The document can be customized and modified after downloading, please adjust and use it according to actual needs, thank you! In addition, this shop provides you with various types of practical materials, such as educational essays, diary appreciation, sentence excerpts, ancient poems, classic articles, topic composition, work summary, word parsing, copy excerpts, other materials and so on, want to know different data formats and writing methods, please pay attention!细胞色素P450还原酶和细胞色素P450细胞色素P450(Cytochrome P450,简称CYP)是一类广泛存在于生物体内的酶,它们在代谢物的合成和降解中发挥着至关重要的作用。
药物代谢p450

药物代谢P4501. 引言药物代谢是指在人体内将药物转化为更容易排出体外的代谢产物的过程。
其中,药物代谢酶P450家族(CYP450)起着重要作用。
本文将深入探讨药物代谢P450的机制、影响因素以及与临床相关的应用。
2. 药物代谢P450的机制P450酶是一类存在于内质网膜上的细胞色素酶,它通过氧化反应将药物分子中的非活性碳氢键转化为活性基团,从而使药物具有更高的亲水性和更强的极性。
这些活性基团进一步被结合到内源性或外源性底物上,形成可溶于水的代谢产物。
药物代谢P450主要包括两个阶段:相1反应和相2反应。
相1反应由CYP450酶催化,包括氧化、还原和水解等反应类型。
相2反应则是在相1反应后进行,涉及底物与内源性底物(如葡萄糖醛酸、硫酸盐和甘氨酸)的结合。
3. CYP450家族和药物代谢CYP450家族包括多个亚型,其中CYP1A2、CYP2C9、CYP2D6和CYP3A4是最常见的亚型。
这些亚型在药物代谢中起着重要作用,因为它们参与代谢了大部分药物。
•CYP1A2:参与咖啡因、茶碱等药物的代谢。
•CYP2C9:参与华法林、非甾体类抗炎药等药物的代谢。
•CYP2D6:参与抗精神病药物、β受体阻滞剂等药物的代谢。
•CYP3A4:参与许多药物(如氟西汀、阿司匹林)的代谢。
4. 影响药物代谢P450的因素多种因素可以影响药物在体内的代谢过程,包括遗传因素、环境因素和个体差异等。
4.1 遗传因素不同人群之间存在基因多态性,这些基因变异可能导致对特定药物的代谢能力差异。
例如,CYP2D6基因突变可能导致对某些药物的代谢能力降低,从而增加药物的毒副作用。
4.2 环境因素环境因素如饮食、吸烟和饮酒等也可能对药物代谢产生影响。
其中,某些食物或饮料可能通过抑制或诱导CYP450酶的活性来改变药物代谢速率。
4.3 个体差异每个人的药物代谢能力都存在一定差异,这可能与年龄、性别、肝脏功能和其他疾病状态等因素有关。
例如,婴儿和老年人的药物代谢能力较低,容易发生药物积累。
CYP酶
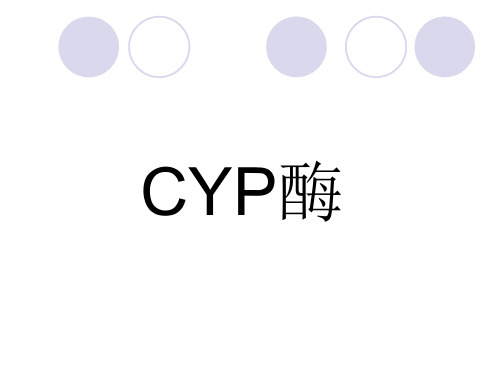
二、香豆素类化合物对细胞色素P450的调控 作用 据报道香豆素类化合物,能选择性抑制 肠壁组织的CYP3A4,而大多数药物是经 CYP3A4代谢,并且肠壁组织的CYP3A4是 药物发生首过效应的主要代谢酶,所以, 服用含香豆素类化合物的中药可以减少许 多联用药物的首过效应而产生不良反应。
三、生物碱类化合物对细胞色素P450的调控 作用 盐酸小檗碱是一种从黄连和黄柏等中药 中提取出的小檗碱盐酸盐,其单独使用或 与环孢素A合用对大鼠肝脏CYP3A1、 CYP2E1基因表达均有明显的抑制作用。吴 茱萸次碱是从吴茱萸中提取的喹啉类生物 碱,体外试验对人脏脏CYPlA2、CYP2C19、 CYP2D6、CYP2E1活性有抑制作用,对 CYP2C9、CYP3A4则无明显抑制作用.
2、酶的抑制 酶的抑制则增加了该酶代谢的药物的浓度,延 长了药理作用时间,并增加了药物的不良反应。 CYP的抑制是引起药物不良反映最常见的原因。 例如特非那定与酮康唑合用时,由于酮康唑是 CYP3A的强效抑制剂药,而特非那定在体内主 要由CYP3A代谢,所以,酮康唑可明显抑制特 非那定的代谢,造成特非那定血药浓度明显升 高,进而引起不良反应。 可用的论文\实验类\可用的\酮康唑对健康成人肝 细胞微粒体细胞色素P450同工酶3A4_1A2活性 的作用.pdf
CYP酶
概述
CYP酶(也称细胞色素氧化酶P450 )是一组结 构和功能相关的超家族基因编码的同工酶. 它们是主要存在于肝脏、肠道中的单加氧酶,多 位于细胞内质网上,是内质网膜上混合功能氧化 酶系统的末端氧化酶。参与内源性物质(如脂肪 酸、维生素、胆酸)的代谢,外源性物质(如药 物)的解毒,前致癌物质(如芳香类物质)的激 活,在药物代谢中发挥重要的作用. 细胞色素P450同工酶是血红蛋白超级家族,称呼 这些为同工酶是由于酶蛋白中所含血红素与CO的 结合体P450-CO在450 nm处有特征性强吸收峰而 得名. P450同工酶认为已存在了350万年,在细菌、真 菌及动植物中均可发现其存在。
p450生物催化羟基机理
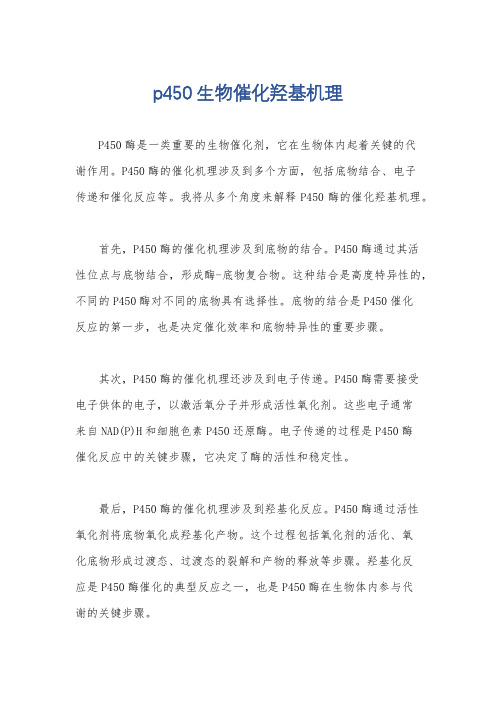
p450生物催化羟基机理
P450酶是一类重要的生物催化剂,它在生物体内起着关键的代
谢作用。
P450酶的催化机理涉及到多个方面,包括底物结合、电子
传递和催化反应等。
我将从多个角度来解释P450酶的催化羟基机理。
首先,P450酶的催化机理涉及到底物的结合。
P450酶通过其活
性位点与底物结合,形成酶-底物复合物。
这种结合是高度特异性的,不同的P450酶对不同的底物具有选择性。
底物的结合是P450催化
反应的第一步,也是决定催化效率和底物特异性的重要步骤。
其次,P450酶的催化机理还涉及到电子传递。
P450酶需要接受
电子供体的电子,以激活氧分子并形成活性氧化剂。
这些电子通常
来自NAD(P)H和细胞色素P450还原酶。
电子传递的过程是P450酶
催化反应中的关键步骤,它决定了酶的活性和稳定性。
最后,P450酶的催化机理涉及到羟基化反应。
P450酶通过活性
氧化剂将底物氧化成羟基化产物。
这个过程包括氧化剂的活化、氧
化底物形成过渡态、过渡态的裂解和产物的释放等步骤。
羟基化反
应是P450酶催化的典型反应之一,也是P450酶在生物体内参与代
谢的关键步骤。
总的来说,P450酶的催化羟基机理涉及底物结合、电子传递和催化反应等多个方面。
这些步骤相互作用,共同促进P450酶的催化活性。
对P450酶催化机理的深入理解对于药物代谢、环境污染修复等领域具有重要意义。
希望这些信息能够满足你的需求。
p450酶系统催化药物反应
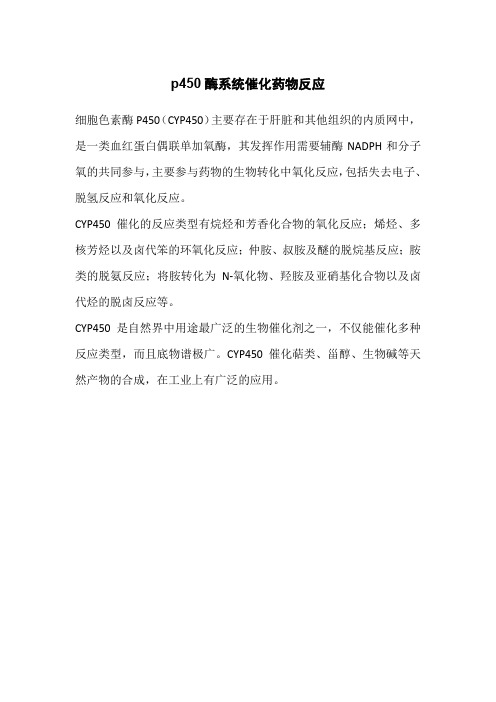
p450酶系统催化药物反应
细胞色素酶P450(CYP450)主要存在于肝脏和其他组织的内质网中,是一类血红蛋白偶联单加氧酶,其发挥作用需要辅酶NADPH和分子氧的共同参与,主要参与药物的生物转化中氧化反应,包括失去电子、脱氢反应和氧化反应。
CYP450催化的反应类型有烷烃和芳香化合物的氧化反应;烯烃、多核芳烃以及卤代笨的环氧化反应;仲胺、叔胺及醚的脱烷基反应;胺类的脱氨反应;将胺转化为N-氧化物、羟胺及亚硝基化合物以及卤代烃的脱卤反应等。
CYP450是自然界中用途最广泛的生物催化剂之一,不仅能催化多种反应类型,而且底物谱极广。
CYP450催化萜类、甾醇、生物碱等天然产物的合成,在工业上有广泛的应用。
细胞色素P450代谢酶的结构与生物学意义
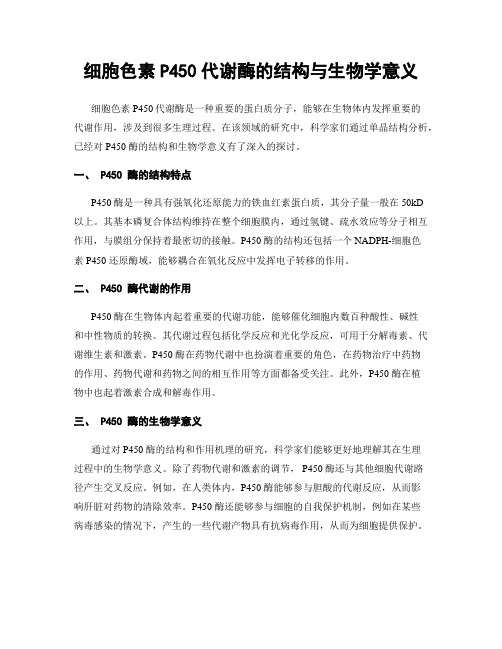
细胞色素P450代谢酶的结构与生物学意义细胞色素P450代谢酶是一种重要的蛋白质分子,能够在生物体内发挥重要的代谢作用,涉及到很多生理过程。
在该领域的研究中,科学家们通过单晶结构分析,已经对 P450 酶的结构和生物学意义有了深入的探讨。
一、 P450 酶的结构特点P450 酶是一种具有强氧化还原能力的铁血红素蛋白质,其分子量一般在50kD以上。
其基本磷复合体结构维持在整个细胞膜内,通过氢键、疏水效应等分子相互作用,与膜组分保持着最密切的接触。
P450 酶的结构还包括一个 NADPH-细胞色素 P450 还原酶域,能够耦合在氧化反应中发挥电子转移的作用。
二、 P450 酶代谢的作用P450 酶在生物体内起着重要的代谢功能,能够催化细胞内数百种酸性、碱性和中性物质的转换。
其代谢过程包括化学反应和光化学反应,可用于分解毒素、代谢维生素和激素。
P450 酶在药物代谢中也扮演着重要的角色,在药物治疗中药物的作用、药物代谢和药物之间的相互作用等方面都备受关注。
此外,P450 酶在植物中也起着激素合成和解毒作用。
三、 P450 酶的生物学意义通过对 P450 酶的结构和作用机理的研究,科学家们能够更好地理解其在生理过程中的生物学意义。
除了药物代谢和激素的调节, P450 酶还与其他细胞代谢路径产生交叉反应。
例如,在人类体内,P450 酶能够参与胆酸的代谢反应,从而影响肝脏对药物的清除效率。
P450 酶还能够参与细胞的自我保护机制,例如在某些病毒感染的情况下,产生的一些代谢产物具有抗病毒作用,从而为细胞提供保护。
总的来说, P450 酶其厂生化性质和功能十分复杂,涉及到许多生理过程和代谢途径。
其结构、代谢作用以及生物学意义的研究成果,不仅为药物治疗提供了一定的理论支持,也有助于人们对自身生物化学代谢系统的深入认识。
p450氧化还原酶试剂
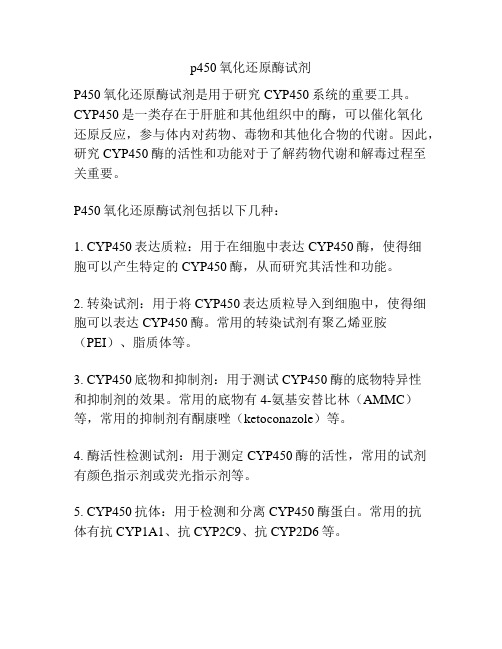
p450氧化还原酶试剂
P450氧化还原酶试剂是用于研究CYP450系统的重要工具。
CYP450是一类存在于肝脏和其他组织中的酶,可以催化氧化
还原反应,参与体内对药物、毒物和其他化合物的代谢。
因此,研究CYP450酶的活性和功能对于了解药物代谢和解毒过程至关重要。
P450氧化还原酶试剂包括以下几种:
1. CYP450表达质粒:用于在细胞中表达CYP450酶,使得细
胞可以产生特定的CYP450酶,从而研究其活性和功能。
2. 转染试剂:用于将CYP450表达质粒导入到细胞中,使得细胞可以表达CYP450酶。
常用的转染试剂有聚乙烯亚胺(PEI)、脂质体等。
3. CYP450底物和抑制剂:用于测试CYP450酶的底物特异性
和抑制剂的效果。
常用的底物有4-氨基安替比林(AMMC)等,常用的抑制剂有酮康唑(ketoconazole)等。
4. 酶活性检测试剂:用于测定CYP450酶的活性,常用的试剂有颜色指示剂或荧光指示剂等。
5. CYP450抗体:用于检测和分离CYP450酶蛋白。
常用的抗
体有抗CYP1A1、抗CYP2C9、抗CYP2D6等。
以上是常见的P450氧化还原酶试剂,可以根据需要选择适合的试剂来进行相关研究。
p450的co差光谱法原理

细胞色素P450(cytochrome P450,CYP450)是一种广泛存在于生物体内的酶,具有重要的代谢作用。
CO差光谱法是一种常用的CYP450含量测定方法,其原理如下:
1. 还原型细胞色素P450(cytochrome c reductase-reduced P450,rP450)可与一氧化碳(CO)结合,形成CO-rP450复合物,在波长450nm处呈现最大吸收峰。
因此,可以应用示差光谱法测定其含量。
2. 在实验中,首先将还原型细胞色素P450加入样品中,并加入还原剂(如NADPH)和细胞色素c还原酶,使其还原为氧化型细胞色素P450(cytochrome c oxidase-oxidized P450,oP450)。
3. 然后,将样品中的氧气(O2)去除,加入CO,使其与氧化型细胞色素P450结合,形成CO-oP450复合物,并释放还原型细胞色素P450。
4. 最后,使用紫外分光光度计测量样品中450nm处的吸光度,并通过计算吸光度差(ΔA)来计算还原型细胞色素P450的含量。
ΔA=A450-A490,其中A450和A490分别表示CO-oP450复合物和CO-rP450复合物的吸光度。
总之,CO差光谱法利用了还原型细胞色素P450与CO结合后呈现出的最大吸收峰,通过测量吸光度差来计算其含量。
该方法操作简便、快速、灵敏度高,适用于多种生物样品的CYP450含量测定。
细胞色素p450酶的特点
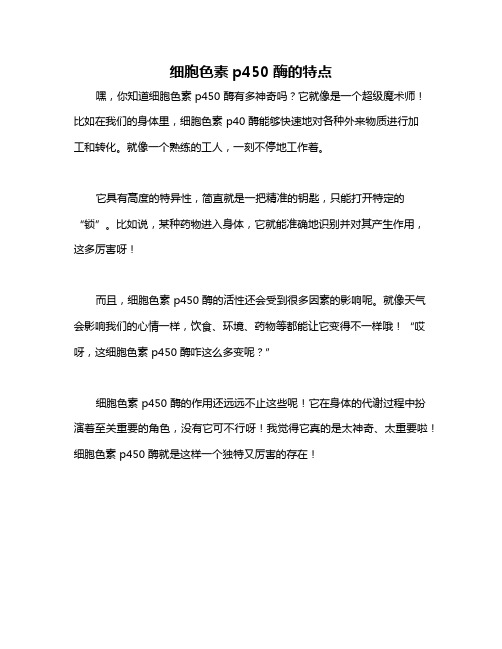
细胞色素p450酶的特点
嘿,你知道细胞色素 p450 酶有多神奇吗?它就像是一个超级魔术师!比如在我们的身体里,细胞色素 p40 酶能够快速地对各种外来物质进行加
工和转化。
就像一个熟练的工人,一刻不停地工作着。
它具有高度的特异性,简直就是一把精准的钥匙,只能打开特定的“锁”。
比如说,某种药物进入身体,它就能准确地识别并对其产生作用,这多厉害呀!
而且,细胞色素 p450 酶的活性还会受到很多因素的影响呢。
就像天气会影响我们的心情一样,饮食、环境、药物等都能让它变得不一样哦!“哎呀,这细胞色素 p450 酶咋这么多变呢?”
细胞色素 p450 酶的作用还远远不止这些呢!它在身体的代谢过程中扮演着至关重要的角色,没有它可不行呀!我觉得它真的是太神奇、太重要啦!细胞色素 p450 酶就是这样一个独特又厉害的存在!。
植物抗病 p450酶
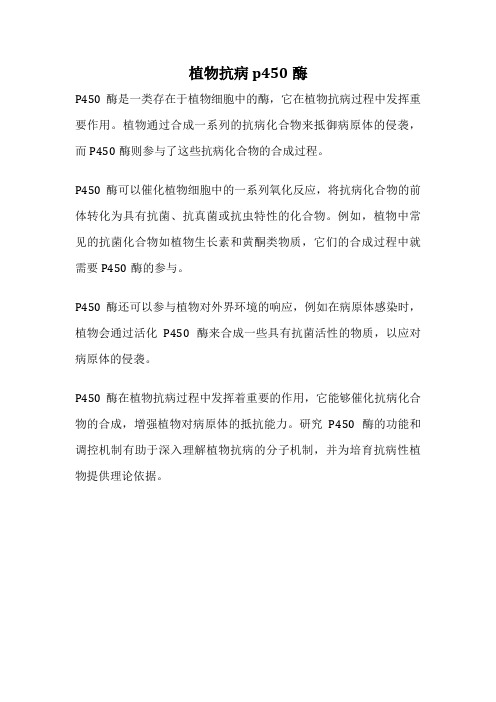
植物抗病 p450酶
P450酶是一类存在于植物细胞中的酶,它在植物抗病过程中发挥重要作用。
植物通过合成一系列的抗病化合物来抵御病原体的侵袭,而P450酶则参与了这些抗病化合物的合成过程。
P450酶可以催化植物细胞中的一系列氧化反应,将抗病化合物的前体转化为具有抗菌、抗真菌或抗虫特性的化合物。
例如,植物中常见的抗菌化合物如植物生长素和黄酮类物质,它们的合成过程中就需要P450酶的参与。
P450酶还可以参与植物对外界环境的响应,例如在病原体感染时,植物会通过活化P450酶来合成一些具有抗菌活性的物质,以应对病原体的侵袭。
P450酶在植物抗病过程中发挥着重要的作用,它能够催化抗病化合物的合成,增强植物对病原体的抵抗能力。
研究P450酶的功能和调控机制有助于深入理解植物抗病的分子机制,并为培育抗病性植物提供理论依据。
p450酶结构
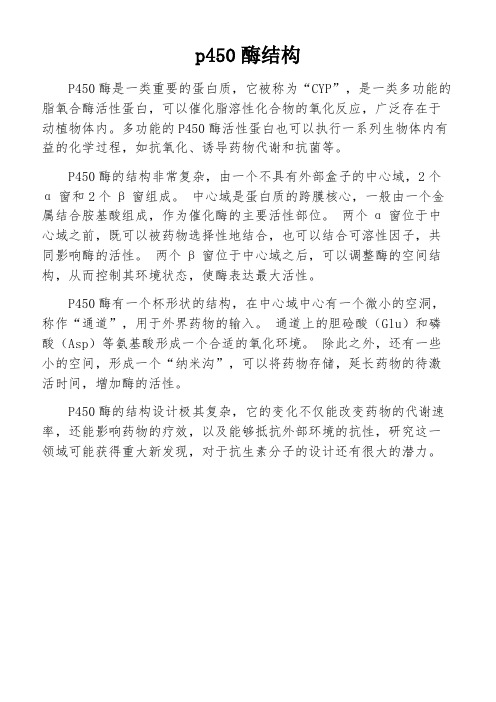
p450酶结构
P450酶是一类重要的蛋白质,它被称为“CYP”,是一类多功能的脂氧合酶活性蛋白,可以催化脂溶性化合物的氧化反应,广泛存在于动植物体内。
多功能的P450酶活性蛋白也可以执行一系列生物体内有益的化学过程,如抗氧化、诱导药物代谢和抗菌等。
P450酶的结构非常复杂,由一个不具有外部盒子的中心域,2个α窗和2个β窗组成。
中心域是蛋白质的跨膜核心,一般由一个金属结合胺基酸组成,作为催化酶的主要活性部位。
两个α窗位于中心域之前,既可以被药物选择性地结合,也可以结合可溶性因子,共同影响酶的活性。
两个β窗位于中心域之后,可以调整酶的空间结构,从而控制其环境状态,使酶表达最大活性。
P450酶有一个杯形状的结构,在中心域中心有一个微小的空洞,称作“通道”,用于外界药物的输入。
通道上的胆硷酸(Glu)和磷酸(Asp)等氨基酸形成一个合适的氧化环境。
除此之外,还有一些小的空间,形成一个“纳米沟”,可以将药物存储,延长药物的待激活时间,增加酶的活性。
P450酶的结构设计极其复杂,它的变化不仅能改变药物的代谢速率,还能影响药物的疗效,以及能够抵抗外部环境的抗性,研究这一领域可能获得重大新发现,对于抗生素分子的设计还有很大的潜力。
p450酶催化机理
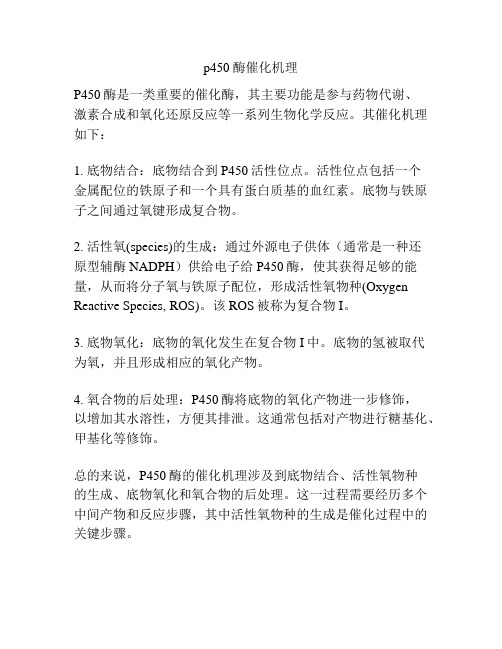
p450酶催化机理
P450酶是一类重要的催化酶,其主要功能是参与药物代谢、
激素合成和氧化还原反应等一系列生物化学反应。
其催化机理如下:
1. 底物结合:底物结合到P450活性位点。
活性位点包括一个
金属配位的铁原子和一个具有蛋白质基的血红素。
底物与铁原子之间通过氧键形成复合物。
2. 活性氧(species)的生成:通过外源电子供体(通常是一种还
原型辅酶NADPH)供给电子给P450酶,使其获得足够的能量,从而将分子氧与铁原子配位,形成活性氧物种(Oxygen Reactive Species, ROS)。
该ROS被称为复合物I。
3. 底物氧化:底物的氧化发生在复合物I中。
底物的氢被取代
为氧,并且形成相应的氧化产物。
4. 氧合物的后处理:P450酶将底物的氧化产物进一步修饰,
以增加其水溶性,方便其排泄。
这通常包括对产物进行糖基化、甲基化等修饰。
总的来说,P450酶的催化机理涉及到底物结合、活性氧物种
的生成、底物氧化和氧合物的后处理。
这一过程需要经历多个中间产物和反应步骤,其中活性氧物种的生成是催化过程中的关键步骤。
细胞色素p450名词解释
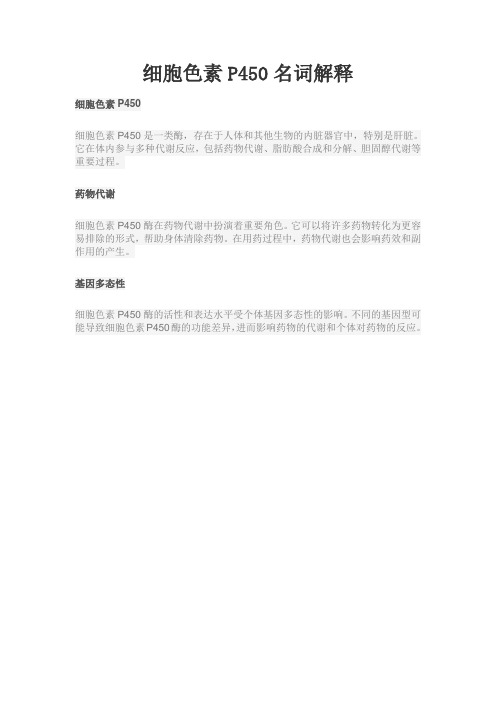
细胞色素P450名词解释
细胞色素P450
细胞色素P450是一类酶,存在于人体和其他生物的内脏器官中,特别是肝脏。
它在体内参与多种代谢反应,包括药物代谢、脂肪酸合成和分解、胆固醇代谢等重要过程。
药物代谢
细胞色素P450酶在药物代谢中扮演着重要角色。
它可以将许多药物转化为更容易排除的形式,帮助身体清除药物。
在用药过程中,药物代谢也会影响药效和副作用的产生。
基因多态性
细胞色素P450酶的活性和表达水平受个体基因多态性的影响。
不同的基因型可能导致细胞色素P450酶的功能差异,进而影响药物的代谢和个体对药物的反应。
细胞色素P450的功能和调节
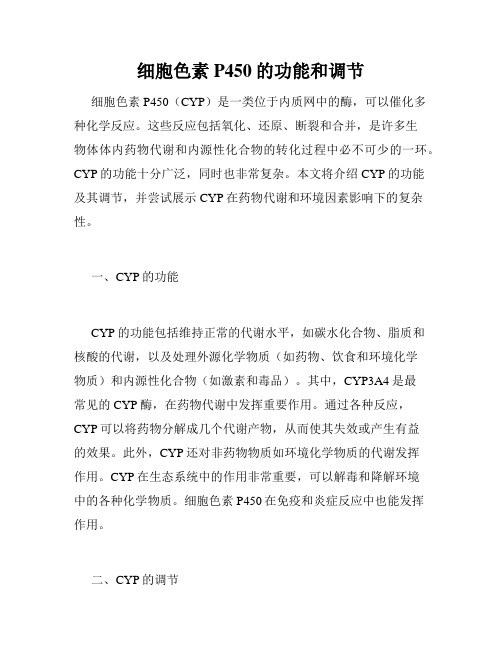
细胞色素P450的功能和调节细胞色素P450(CYP)是一类位于内质网中的酶,可以催化多种化学反应。
这些反应包括氧化、还原、断裂和合并,是许多生物体体内药物代谢和内源性化合物的转化过程中必不可少的一环。
CYP的功能十分广泛,同时也非常复杂。
本文将介绍CYP的功能及其调节,并尝试展示CYP在药物代谢和环境因素影响下的复杂性。
一、CYP的功能CYP的功能包括维持正常的代谢水平,如碳水化合物、脂质和核酸的代谢,以及处理外源化学物质(如药物、饮食和环境化学物质)和内源性化合物(如激素和毒品)。
其中,CYP3A4是最常见的CYP酶,在药物代谢中发挥重要作用。
通过各种反应,CYP可以将药物分解成几个代谢产物,从而使其失效或产生有益的效果。
此外,CYP还对非药物物质如环境化学物质的代谢发挥作用。
CYP在生态系统中的作用非常重要,可以解毒和降解环境中的各种化学物质。
细胞色素P450在免疫和炎症反应中也能发挥作用。
二、CYP的调节CYP可以在许多不同的方面进行调节。
其中,最常见的是体内的调节。
重要的调节因子包括维生素D、激素、细胞因子和环境污染物。
此外,钙离子在CYP调节中也发挥重要作用。
酶的活性可能受到磷酸化、去磷酸化和乙酰化的影响。
因此,蛋白激酶、磷酸酰酶、组蛋白去乙酰化酶以及其他修饰酶可能在CYP调节中发挥作用。
环境因素也是CYP调节的重要因素。
药物、天然产物和环境污染物都被证明能够对CYP酶发挥活性或致使其受到抑制。
例如,药物产生的代谢产物会与CYP结合并抑制酶的活性。
环境污染物也可以影响CYP的活性,有时候这些物质被称为“雌激素样物质”,因为它们可以增加生物体中的雌激素水平,并可能导致一系列不良反应,如激素依赖性癌症。
三、药物交互作用药物交互作用是指两种或更多药物在某种方面相互干扰。
CYP酶参与药物代谢的过程中,药物交互作用必须得到重视,这是因为在治疗过程中,常常需要一些药物的联合使用,这些药物之间可能存在相互干扰的关系。
水稻p450基因

水稻p450基因
水稻p450基因是水稻基因中的一类,属于细胞色素p450酶家族的成员。
p450基因家族是一类编码酶蛋白的基因家族,参与多种生物化学反应,包括代谢内源化合物和外源物质,如毒物、激素和药物等。
在水稻中,p450基因参与了许多重要的生理过程,例如激素合成、次生代谢物合成和抗性反应等。
水稻p450基因的功能主要包括催化酶反应、代谢物合成和分解、维持内源化合物平衡等。
通过调控水稻p450基因的表达水平,可以影响水稻的生长发育、抵抗病虫害和适应环境变化等方面的性状。
研究水稻p450基因的表达和功能有助于深入理解水稻的生物代谢途径和相关生理过程,并为水稻基因改良和培育优良品种提供理论依据。
p450酶系名词解释
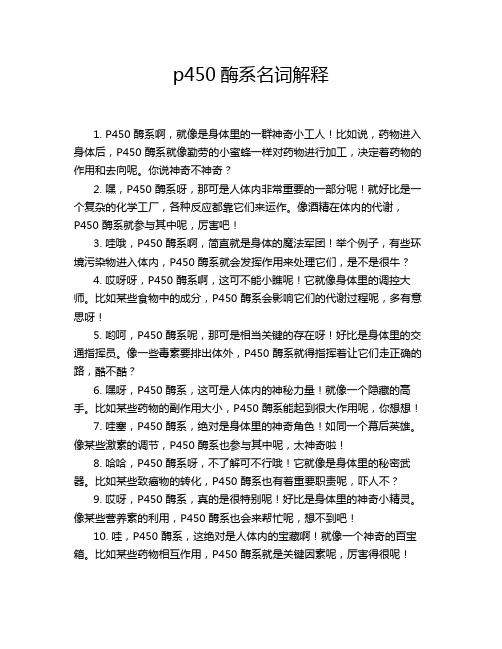
p450酶系名词解释1. P450 酶系啊,就像是身体里的一群神奇小工人!比如说,药物进入身体后,P450 酶系就像勤劳的小蜜蜂一样对药物进行加工,决定着药物的作用和去向呢。
你说神奇不神奇?2. 嘿,P450 酶系呀,那可是人体内非常重要的一部分呢!就好比是一个复杂的化学工厂,各种反应都靠它们来运作。
像酒精在体内的代谢,P450 酶系就参与其中呢,厉害吧!3. 哇哦,P450 酶系啊,简直就是身体的魔法军团!举个例子,有些环境污染物进入体内,P450 酶系就会发挥作用来处理它们,是不是很牛?4. 哎呀呀,P450 酶系啊,这可不能小瞧呢!它就像身体里的调控大师。
比如某些食物中的成分,P450 酶系会影响它们的代谢过程呢,多有意思呀!5. 哟呵,P450 酶系呢,那可是相当关键的存在呀!好比是身体里的交通指挥员。
像一些毒素要排出体外,P450 酶系就得指挥着让它们走正确的路,酷不酷?6. 嘿呀,P450 酶系,这可是人体内的神秘力量!就像一个隐藏的高手。
比如某些药物的副作用大小,P450 酶系能起到很大作用呢,你想想!7. 哇塞,P450 酶系,绝对是身体里的神奇角色!如同一个幕后英雄。
像某些激素的调节,P450 酶系也参与其中呢,太神奇啦!8. 哈哈,P450 酶系呀,不了解可不行哦!它就像是身体里的秘密武器。
比如某些致癌物的转化,P450 酶系也有着重要职责呢,吓人不?9. 哎呀,P450 酶系,真的是很特别呢!好比是身体里的神奇小精灵。
像某些营养素的利用,P450 酶系也会来帮忙呢,想不到吧!10. 哇,P450 酶系,这绝对是人体内的宝藏啊!就像一个神奇的百宝箱。
比如某些药物相互作用,P450 酶系就是关键因素呢,厉害得很呢!我觉得 P450 酶系真的太重要了,我们应该多了解它,这样才能更好地保护我们的身体呀!。
细胞色素P450基因家族的功能与应用

细胞色素P450基因家族的功能与应用细胞色素P450基因家族,简称P450,是一类在生物体内起着重要代谢作用的基因家族。
它们存在于多种生物体中,包括微生物、植物和动物,特别是在哺乳动物中更是广泛存在。
细胞色素P450基因家族具有多种功能,例如药物代谢、毒物解毒、激素合成等,因此在药物开发和环境保护等领域都有着广泛的应用。
P450基因家族的命名方式是由其吸收最大波长(max)在450纳米处的细胞色素谱线命名而来。
根据P450基因家族的基序差异和基因簇的相似度,目前已经鉴定出了超过50个家族和多种亚型,其中以CYP1、CYP2和CYP3三个家族的细胞色素P450酶在药物和毒物代谢中发挥着重要作用。
一、药物代谢细胞色素P450酶在药物代谢中发挥了重要作用,它们能够代谢掉各种不同种类的药物并且调节它们在体内的水平。
不同的药物在体内可能会产生副作用或毒性,这时P450酶会降解它们,减轻它们的不良影响。
如CYP3A4是人体内最常见的细胞色素P450酶之一,其在肝脏中负责代谢70%以上的药物,这也就是为什么许多药物都需要通过肝脏代谢来达到治疗效果。
因此,研究P450基因家族在药物合成和代谢中的调节机制,对于药物研发和治疗方案的优化具有重要意义。
二、毒物代谢除了药物代谢之外,细胞色素P450酶在毒物代谢中也发挥着重要作用,它们能够代谢掉体内吸入或摄入的毒物,防止毒物对身体造成更严重的危害。
这样,细胞色素P450基因家族也成为了环境保护中的重要工具。
例如,CYP1A1酶可以代谢类固醇激素、多环芳烃等环境污染物,同时也可以代谢大量的致癌物。
因此,了解P450基因家族在毒物代谢中的调节机制,有助于减轻环境污染对人类健康的威胁。
三、激素合成除了药物代谢和毒物代谢之外,细胞色素P450酶还参与了激素合成的过程。
大多数激素、荷尔蒙和其他生物活性化合物都需要通过细胞色素P450酶的参与才能被合成或生物活化。
例如,CYP19A1酶是人体内合成雌激素的主要酶,在正常人体内合成的雌激素中就比较依赖于细胞色素P450酶的催化作用。
细胞色素 p450组成结构及化学式
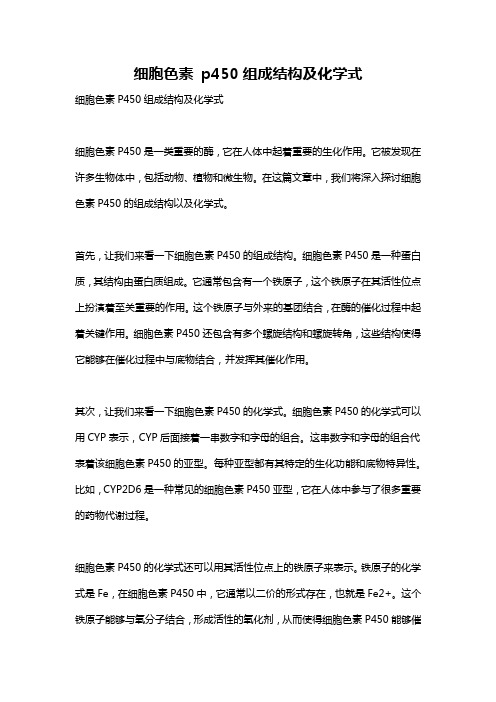
细胞色素p450组成结构及化学式细胞色素P450组成结构及化学式细胞色素P450是一类重要的酶,它在人体中起着重要的生化作用。
它被发现在许多生物体中,包括动物、植物和微生物。
在这篇文章中,我们将深入探讨细胞色素P450的组成结构以及化学式。
首先,让我们来看一下细胞色素P450的组成结构。
细胞色素P450是一种蛋白质,其结构由蛋白质组成。
它通常包含有一个铁原子,这个铁原子在其活性位点上扮演着至关重要的作用。
这个铁原子与外来的基团结合,在酶的催化过程中起着关键作用。
细胞色素P450还包含有多个螺旋结构和螺旋转角,这些结构使得它能够在催化过程中与底物结合,并发挥其催化作用。
其次,让我们来看一下细胞色素P450的化学式。
细胞色素P450的化学式可以用CYP表示,CYP后面接着一串数字和字母的组合。
这串数字和字母的组合代表着该细胞色素P450的亚型。
每种亚型都有其特定的生化功能和底物特异性。
比如,CYP2D6是一种常见的细胞色素P450亚型,它在人体中参与了很多重要的药物代谢过程。
细胞色素P450的化学式还可以用其活性位点上的铁原子来表示。
铁原子的化学式是Fe,在细胞色素P450中,它通常以二价的形式存在,也就是Fe2+。
这个铁原子能够与氧分子结合,形成活性的氧化剂,从而使得细胞色素P450能够催化多种生物化学反应。
最后,让我们来总结一下细胞色素P450的组成结构及化学式。
细胞色素P450是一种含有铁原子的蛋白质酶,其结构复杂多样,具有多个螺旋结构和螺旋转角。
它的化学式可以用CYP表示,也可以用其活性位点上的铁原子来表示。
细胞色素P450在人体中发挥着重要的生化作用,特别是参与了许多药物代谢过程。
希望通过本文的介绍,读者能对细胞色素P450的组成结构及化学式有更深入的了解。
光驱动 催化 p450
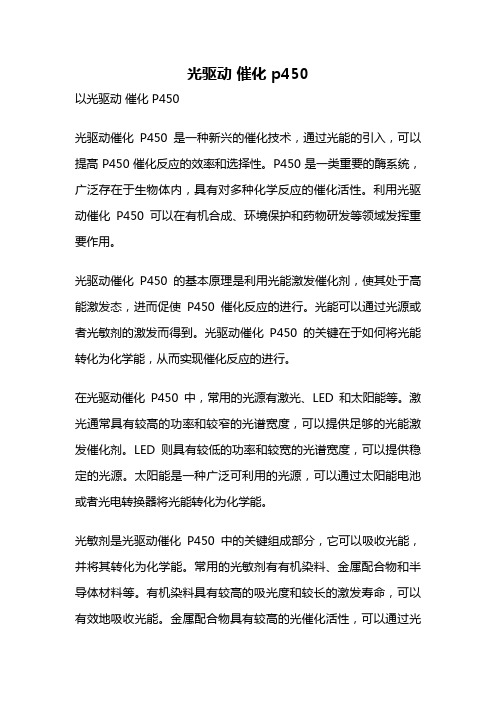
光驱动催化 p450以光驱动催化 P450光驱动催化P450是一种新兴的催化技术,通过光能的引入,可以提高P450催化反应的效率和选择性。
P450是一类重要的酶系统,广泛存在于生物体内,具有对多种化学反应的催化活性。
利用光驱动催化P450可以在有机合成、环境保护和药物研发等领域发挥重要作用。
光驱动催化P450的基本原理是利用光能激发催化剂,使其处于高能激发态,进而促使P450催化反应的进行。
光能可以通过光源或者光敏剂的激发而得到。
光驱动催化P450的关键在于如何将光能转化为化学能,从而实现催化反应的进行。
在光驱动催化P450中,常用的光源有激光、LED和太阳能等。
激光通常具有较高的功率和较窄的光谱宽度,可以提供足够的光能激发催化剂。
LED则具有较低的功率和较宽的光谱宽度,可以提供稳定的光源。
太阳能是一种广泛可利用的光源,可以通过太阳能电池或者光电转换器将光能转化为化学能。
光敏剂是光驱动催化P450中的关键组成部分,它可以吸收光能,并将其转化为化学能。
常用的光敏剂有有机染料、金属配合物和半导体材料等。
有机染料具有较高的吸光度和较长的激发寿命,可以有效地吸收光能。
金属配合物具有较高的光催化活性,可以通过光氧化还原反应转化光能。
半导体材料具有较高的光电转换效率,可以将光能转化为电能或化学能。
光驱动催化P450的应用十分广泛。
在有机合成中,光驱动催化P450可以替代传统的热驱动催化反应,提高反应速率和产率。
在环境保护中,光驱动催化P450可以利用太阳能等可再生能源,实现有害物质的高效降解和废水的处理。
在药物研发中,光驱动催化P450可以辅助药物合成和代谢研究,为新药的研发提供技术支持。
光驱动催化P450的发展还面临一些挑战。
首先,光驱动催化P450需要精确控制光能的输入和输出,以避免能量损失和副反应的发生。
其次,光驱动催化P450需要克服光敏剂的稳定性和寿命问题,以提高催化效率和使用寿命。
最后,光驱动催化P450需要与传统的催化技术相结合,以实现更高效的催化反应。
- 1、下载文档前请自行甄别文档内容的完整性,平台不提供额外的编辑、内容补充、找答案等附加服务。
- 2、"仅部分预览"的文档,不可在线预览部分如存在完整性等问题,可反馈申请退款(可完整预览的文档不适用该条件!)。
- 3、如文档侵犯您的权益,请联系客服反馈,我们会尽快为您处理(人工客服工作时间:9:00-18:30)。
PAPER /dalton |Dalton TransactionsTheoretical study of N -dealkylation of N -cyclopropyl-N -methylanilinecatalyzed by cytochrome P450:insight into the origin of the regioselectivity†Dongmei Li,a ,b Yong Wang,a Chuanlu Yang c and Keli Han*aReceived 25th June 2008,Accepted 1st October 2008First published as an Advance Article on the web 19th November 2008DOI:10.1039/b810767jThe mechanism of N -dealkylation of N -cyclopropyl-N -methylaniline (3)catalyzed by cytochrome P450(P450)was investigated using density functional theory.This reaction involves two steps.The first one is a C a –H hydroxylation on the N -substituent to form a carbinolaniline complex,and the second is a decomposition of the carbinolaniline to yield cyclopropanone (or formaldehyde)and N -methylaniline (or N -cyclopropylaniline).Our calculations demonstrate that the first step proceeds in a spin-selective mechanism (SSM),mostly on the low-spin (LS)doublet state.The rate-limiting C a –H activation is an isotope-sensitive hydrogen atom transfer (HAT)step.The environmental effect switches the regioselectivity of this reaction from a competition between N -decyclopropylation andN -demethylation to a clear preference for N -demethylation.This preference is consistent with former experimental studies.However,it is not in accord with the normal D E-BDE correlation since the BDE of C a –H on the methyl group is higher than that on the cyclopropyl group.Insight into the origin of the preference for N -demethylation reveals that tertiary amine 3is different from normal hydrocarbons,possessing a unique p Ph -p C-N conjugated system.The electron delocalization effect of the p Ph -p C-N conjugated system in 3makes the transition state pose a polar character,and the bulk polarity and hydrogen bonding capability of the protein pocket can exert a remarkable effect on the regioselectivity of N -dealkylation of 3.Decomposition of carbinolaniline is a water-assisted proton-transfer process in the nonenzymatic environment.The ring-intact cyclopropanone formed in the reaction sheds some light on the inability of 3to inactivate P450during its N -decyclopropylation.IntroductionCytochrome P450(P450)is a family of heme-monooxygenases found in most forms of life including bacteria,plants,and mammals.Due to its key role in many biochemical processes,the mechanism of P450-catalyzed reactions has been extensively studied.1–10One of the most intriguing reactions is N -dealkylation of alkylamine,which has been of great interest and debated for many years.Two alternative mechanisms have survived the years of discourse.One is a formal hydroxylation of a C–H bond on the carbon adjacent to the heteroatom (HAT,hydrogen atom transfer),11–14the other is a one-electron oxidation of the heteroatom itself (SET,single electron transfer).5,15The cyclopropylamine structure is found in numerous natural products,drugs and drug candidates,many of which undergo P450-catalyzed N -dealkylation with loss of the cyclopropyl group and other acyclic alkyl groups.Some cyclopropylamines are also suicide substrates for P450.16–18The cyclopropyl group’s unique electronic structure compared to ordinary aliphatic groups 19and unique ability to inactivate P45016–18promoted cyclopropylaminesa State Key Laboratory of Molecular Reaction Dynamics,Dalian Institute of Chemical Physics,Chinese Academy of Sciences,Dalian 116023,China.E-mail:klhan@;Fax:+86-411-84675584;Tel:+86-411-84379293bGraduate School of the Chinese Academy of Sciences,Beijing 100039,China cDepartment of Physics,Ludong University,Yantai 264025,China†Electronic supplementary information (ESI)available:Tables S1–S5:SCF energies,Mulliken charge and spin densities,and data for KIE calculations.See DOI:10.1039/b810767jbeing widely applied as probes of P450and other oxidative enzymes in many mechanistic studies 20–30involved in the “HAT-SET controversy”.The HAT mechanism was early and widely accepted on the observation that the alkyl group lost from an ordinary amine during N -dealkylation appeared as an aldehyde or ketone,which arises from the decomposition of a carbinolamine intermediate.31,32Hanzlik once invoked this mechanism to explain the observation that N -benzyl-N -cyclopropylamine (1)inactivated P450as it underwent P450-catalyzed oxidation.16However,the subsequent observation that N -benzyl-N -(1¢-methylcyclopropyl)amine (2),the 1¢-methyl derivative of 1,which lacks an a -H,also inactivated P450nearly as efficiently as 117,18challenged the HAT mechanism and proposed the SET mechanism.Since then,both mechanisms have been debated by many different authors.However,for many years,the fate of the cyclopropyl group lost during N -dealky-lation and the nature of the reactive intermediate involved in the inactivation of P450remained obscure.More recently,Hanzlik and co-workers performed product studies on the oxidation of cy-clopropylamines by P450and other enzymes using dual [14C/13C]labeling NMR.They first reported that oxidation of N -cyclo-propyl-N -methylaniline (3)by horseradish peroxidase,a well-known SET oxidant,led exclusively to ring-opened products.21,22In sharp contrast,P450-catalyzed oxidation of 3led to ring-intact metabolite cyclopropanone hydrate and no ring-opened products,and 3did not inactivate P450.23The characterization of the cyclopropanone hydrate provided a direct evidence for the HAT mechanism and ruled out the SET mechanism.To helpD o w n l o a d e d b y D A L I A N U N I VE R S I T Y OF T E C H N O L OG Y o n 31 J u l y 2010P u b l i s h e d o n 19 N o v e m b e r 2008 o n h t t p ://p u b s .r s c .o r g | d o i :10.1039/B 810767Jreconcile the different behaviors of 3with SET versus HAT,Cerny and Hanzlik undertook a detailed exploration of the interaction of 1with P450,both as a substrate and as an inactivator.24They reported for the first time that 3-hydroxypropionaldehyde (3HP),the long anticipated “signature metabolite”for SET oxidation of a cyclopropylamine,was indeed a major metabolite of 1.They also found that N -benzyl-N -cyclopropyl-N -methylamine (4)did not inactivate P450and did not give rise to 3HP without first undergo-ing N -demethylation to 1.Thus,they suggested that HAT at C a –H bonds in secondary and tertiary cyclopropylamines (e.g.,1,4and 3)led to nonrearranged carbinolamine intermediates and thereby to “ordinary”N -dealkylation products including the ring-intact cyclopropanone hydrate.Alternatively,HAT at the N–H bond (N-HAT)of secondary cyclopropylamines (e.g.,1)gave a neutral aminyl radical,which could undergo rapid ring-opening leading either to enzyme inactivation or 3HP formation (Scheme 1).Therefore,it’s necessary for us to model the N -dealkylation of cyclopropylamines and identify the fate of the cyclopropyl group that is lost during the reaction in the aspect of calculation,which can shed light on the formation of cyclopropanone hydrate and 3HP or the enzyme inactivation.Herein,we use density functional theory (DFT)to focus on the HAT of tertiary cyclopropylamine 3,which leads to the ring-intact cyclopropanone hydrate.The N-HAT of secondary cyclopropylamine 1,which leads to the ring-opened 3HP and inactivation of the enzyme is underway.Scheme 1Proposed mechanisms for interaction of 1,3,4with P450.Many DFT studies have been performed to elucidate the mechanism of C–H hydroxylation.9,10An excellent linear corre-lation between the energy barrier (D E)of HAT by the active species of P450(Compound I,Cpd I in brief)and the C–H bond dissociation energy (BDE)was established for a series of hydrocarbons.33Experimental studies showed that the barrier ofHAT by metal-oxo complexes or non-heme iron(IV)-oxo catalysts exhibited a linear correlation with the C–H bond energy as well as with the strength of the newly formed O–H bond.34,35DFT calculations on the energies of HAT were demonstrated to be able to predict the regioselectivity of substrate metabolism.36While rate constants and selectivities for HAT from normal hydrocarbons were generally expected to parallel the strength of the C–H bond,this trend was not observed for tertiary amines.Hydrogen abstractions from tertiary amines by the t -butoxyl radical (t BuO ∑,which had been used as the chemical model for P450)were reported to be entropy controlled,and the activation energy did not vary significantly with the C–H BDE.37,38Moreover,product analysis by Shaffer et al.23revealed a preference for N -demethylation over N -decyclopropylation in the metabolism of 3by P450.Bhakta and Wimalasena 25,26also observed a high product ratio of p -Cl-N -cyclopropylaniline to p -Cl-methylaniline in the N -dealkylation of p -Cl-3,although the C a –H bond strength on the cyclopropyl group was weaker than that on the methyl group in 3(or p -Cl-3).Earlier theoretical studies on the hydroxylation mechanism of alkanes revealed a two-state reactivity (TSR)scenario,39–43originating from the high-spin (HS)quartet and low-spin (LS)doublet states of Cpd I.However,recent work by Li et al.44indicated that C a –H hydroxylation of N,N -dimethylaniline (5)was in a spin-selective manner (SSM).Moreover,the results of Wang et al.45showed that the mechanism switched from SSM to TSR in the C a –H hydroxylation of a series of p -substituted 5.From the above description,we can see that the mechanism of 3N -dealkylation poses some intriguing puzzles.First,since the D E-BDE correlation is predictive for normal hydrocarbons,33,46why do the activation energies for tertiary amines not parallel the C–H BDEs 37,38and why do experiments 23,25,26show an observation that N -demethylation is preferred over N -decyclopropylation?Is this an intrinsic property of the substrate undergoing N -dealkylation?Second,DFT calculations show significant differences between the C–H hydroxylation of 5and alkanes,44different p -substituted 5also possess different mechanisms,45so what kind of HAT mecha-nism does 3N -dealkylation proceed by,TSR or SSM?The present work tries to answer the aforementioned questions by performing DFT calculations on N -dealkylation of 3by Cpd I and provides some essential and complementary insights into the experiments.Computational methodAll DFT calculations presented here were performed using the Gaussian03program 47and employed the unrestricted hybrid density functional method UB3L YP .48–51We employed an iron-oxo porphyrin without side chains and a thiolate axial ligand (SH -)to model Cpd I.41,42,44,45,52–58Geometries for all the species were fully optimized without symmetry constraints.For geometry optimization we used the double-z LACVP basis set 59,60on iron and the 6–31G*basis set on the remaining atoms (B1in brief).Single-point energy calculations were performed with higher basis sets,the triple-z LACV3P+*basis set 59,60on iron and the 6–311+G*basis set on all other atoms (B2in brief).These basis sets have been fully tested and proved to be reliable.9,10,44,45Transition states were ascertained by vibrational analysis with only one imaginary frequency mode.The weak polarization effect of the protein environment and the strong polarization effect of the nonenzymatic environment were modeled using theD o w n l o a d e d b y D A L I A N U N I VE R S I T Y OF T E C H N O L OG Y o n 31 J u l y 2010P u b l i s h e d o n 19 N o v e m b e r 2008 o n h t t p ://p u b s .r s c .o r g | d o i :10.1039/B 810767JPCM solvation method with dielectric constants of e =5.62(chlorobenzene)and e =78.39(water)separately.The effect of hydrogen bonding to the sulfur ligand was mimicked by adding two ammonia molecules to the system which point toward the sulfur of the proximal ligand at a fixed distance of r NH–S =2.660A˚.44,45,52–56,61,62The kinetic isotope effect (KIE)of the C a –H hydroxylation step of the reaction was estimated by replacing hydrogen by deuterium in the substrate and was determined using the frequency data based on two expressions.63Semiclassical KIEs were estimated with the Eyring equation:KIE k k G G RT E HD D H ==()-()ÈÎÍ͢˚˙˙ππexp D D (1)In this equation R is the gas constant and T is the temperature(310.15K).Correction to the semiclassical KIEs using the Wigner model can be achieved by multiplying the KIE E by the tunneling ratio (Q t H /Q t D )as follows:KIE KIE Q Q W E t H t D=¥,,(2)Q h k T t B =+()124u /(3)Here,h is the Planck’s constant,k B is the Boltzmann constant,and u is the value of the imaginary frequency in the transition state.Results and discussionC a –H hydroxylation on the N -substituent of 3by Cpd IThere are two N -dealkylation pathways of 3(Scheme 2,Path A:N -decyclopropylation;Path B:N -demethylation).Fig.1shows the energy profiles for C a –H hydroxylation on the N -substituent of 3by Cpd I.In Path A,C a –H hydroxylation starts with hydrogen transfer from the substrate to the oxygen of Cpd I in both HS and LS states,leading to a pair of transition states (4,2TS 1A ).This C a –H bond activation step is then followed by an O-rebound process,which leads to C a –O bonding and formation of the carbinolaniline–heme complex (4,2PC 1A ).A closer inspection of Fig.1a reveals a few notable points.First,reaction on the LS state is a concerted one,going to the carbinolaniline–heme complex without a distinct rebound step.The radical intermediate on the HS state,4IM 1A ,corresponding to the usual iron-hydroxo/cyclopropyl radical complex,behaves as a shoulder on the potential energy surface and once the cyclopropyl radical snaps out of the weak OH–C a interaction,thesubsequentScheme 2N -dealkylation of 3catalyzed byP450.Fig.1Energy profiles for C a –H hydroxylation (a )on the cyclopropyl group and (b )on the methyl group.Values outside the parentheses are relative potential energies in the gas phase,while the values in parentheses involve corrections due to bulk polarity,NH–S hydrogen bonding,and ZPE.RC:reactant complex;TS:transition state;IM:intermediate;PC:product complex.All values are in kcal/mol relative to the reactant 4RC 1A .rebound process is barrierless.Second,hydrogen-transfer is the rate-determining step in the C a –H hydroxylation process.The activation energies are about 11.2/9.3kcal/mol on HS/LS states,and they decrease to 10.8/7.1kcal/mol when environmental effects and ZPE corrections are taken into account.These barriers are much lower than those of alkane hydroxylation,10,33which are derived at least in part from the weaker C a –H bond of 3.Finally,the lowest-lying route occurs on the LS state,which has a 1.9kcal/mol lower reaction barrier than the HS state.This barrier difference increases to 3.7kcal/mol when the bulk polarity,the NH–S hydrogen bonding and ZPE corrections are added.With this large barrier difference,the reaction will proceed predominantly via the LS state.Therefore,C a –H hydroxylation on the cyclopropyl group of 3proceeds via the SSM mechanism similar to that of 544,45rather than the TSR mechanism similar to that of alkane.39–42,61For Path B,C a –H hydroxylation proceeds in a similar concerted way,an initial hydrogen-transfer process involving two states (i.e.HS and LS)followed by a barrier-free rebound to form the corresponding carbinolaniline–heme complex.The HS/LS activation energies are 11.4/9.7kcal/mol,and they decrease toD o w n l o a d e d b y D A L I A N U N I VE R S I T Y OF T E C H N O L OG Y o n 31 J u l y 2010P u b l i s h e d o n 19 N o v e m b e r 2008 o n h t t p ://p u b s .r s c .o r g | d o i :10.1039/B 810767J10.4/5.8kcal/mol when environmental effects and ZPE correc-tions are added.The large barrier difference between HS and LS states makes the C a –H hydroxylation on the methyl group also proceed in an SSM way,predominantly via the LS state.Thus,it is seen that although these two reaction pathways possess different activation energies and different transition states,the “chemical mechanism”does not change.The transition states in Fig.2are hydrogen-transfer species,having no unusual features other than the very large imagi-nary frequencies.On 4TS 1A /2TS 1A ,the angles of C–H–O are 164.2◦/163.1◦,thus the configurations of the C–H–O portions are almost linear.The structures are seen to involve hydrogen-transfer of the radical type,as are evidenced from the large spin densities (r sub =0.68/-0.54)and the small charges (Q sub =0.09/0.13)on the organic moieties.The transition states of C a –H hydroxylation on the methyl group in Fig.2b,4TS 1B /2TS 1B ,also share linear structures (j C–H–O =173.7/175.3)with large spin densities (r sub =0.65/-0.51)and nearly zero charges (Q sub =0.08/0.12)on the organic moieties.Thus,the C a –H activation steps in Path B are also HAT processes.The geometric data in Fig.2alsosuggestFig.2Optimized structures for the C a –H bond activation transition states,4,2TS 1A and 4,2TS 1B .Values outside the parentheses correspond to 4TS 1,while the values in parentheses correspond to 2TS 1.Beside the structures,we indicate the imaginary frequencies of the TS 1species,the spin densities on the organic moiety (r sub )and the migrating H-atom (r H ),and the charge on the organic moiety (Q sub ).that the source of the barrier difference between HS and LS states can be attributed to the degrees of C a –H bond cleavage in the TS 1species.In 2TS 1A and 2TS 1B ,the hydrogen atoms are closer tothe carbon atoms (r C–H,A =1.248A˚,r C–H,B =1.228A ˚)than to the oxygen atoms (r O–H,A =1.310A˚,r O–H,B =1.368A ˚)and are,therefore,more reactant-like in character.On the contrary,the 4TS 1A and 4TS 1B possess more product-like geometries with r C–H,A =1.333A˚(r C–H,B =1.320A˚)and r O–H,A =1.219A ˚(r C–H,B =1.249A ˚).This means that the degrees of C a –H bond cleavage are larger in 4TS 1A and 4TS 1B than those in 2TS 1A and 2TS 1B .Indeed,the barriers for C a –H hydroxylation on the two states reflect the difference in the degrees of C–H bond cleavage in the TS 1species.Fig.3shows the effects of the environment on the relative energies of the transition states for C a –H bond activation.We can see that in the gas phase,the HS transition states are higher than the LS ones by ca.2kcal/mol,and the two pathways are competitive since the energy difference between the two lower lying TS 1species is only 0.4kcal/mol.The bulk polarity of the protein environment increases the energy variations of the four TS 1species and causes a clear preference for the LS transition state in Path B,2TS 1B ,by 1.4kcal/mol.Addition of the NH–S hydrogen bonding effects further accentuates this trend and leads 2TS 1B lower than its counterpart in Path A by 2.0kcal/mol.ZPE corrections decrease the energy gap between 2TS 1A and 2TS 1B ,from 2.0kcal/mol to 1.2kcal/mol,but still predict a preference for Path B.It follows,therefore,that the environmental effects in the protein pocket switch the regioselectivity of the N -dealkylation reaction from a competition between Path A and Path B to a clear preference for Path B.The preference for N -demethylation over N -decyclopropylation is also a common theme in P450-catalyzed cyclopropylamine N -dealkylation ob-served in experimental studies.23,25,26However,the BDE of C a –H on the methyl group is 3.5kcal/mol higher than that on the cyclopropyl group at the UB3L YP/6–311+G*level.Thus,it appears that for P450-catalyzed N -dealkylation of 3,BDE considerations cannot adequately predict which pathway will be preferred,the protein pocket can exert a remarkable effect on the regioselectivity of N -dealkylation of 3.Fig.3Relative energies of the TS 1species at different levels.All values are in kcal/mol relative to 2TS 1A .Insight into the origin of the preference of Path B,which is dominated by the bulk polarity of the hydrophobic heme pocket and the hydrogen bonding to the sulfur ligand of Cpd I,can be gained from Fig.4.Fig.4shows some highly occupied orbitals of 3and TS 1s,along with the dipole moments of the TS 1species.It is seen that in 3the C a –H hybrid orbital is coupled with the lone pair p orbital of N so as to form a p C–N orbital,which isD o w n l o a d e d b y D A L I A N U N I VE R S I T Y OF T E C H N O L OG Y o n 31 J u l y 2010P u b l i s h e d o n 19 N o v e m b e r 2008 o n h t t p ://p u b s .r s c .o r g | d o i :10.1039/B 810767JFig.4Highly occupied orbitals showing electronic delocalization in 3and TS 1species.Below the TS 1species we specify the dipole moments (m )in Debye units.in turn conjugated with the p orbital on the phenyl group and forms a conjugated p Ph -p C–N orbital.The interaction between this p Ph -p C–N conjugated system and Cpd I induces electron flux from the phenyl ring moiety to the C–N moiety,which makes the electron population hold large delocalization and results in the transition state possessing “polar”character.The polar character of the TS 1species is reflected in the dipole moments shown in Fig.4.For each pathway,a larger dipole moment is possessed by the LS transition state.The LS transition state in Path B,2TS 1B ,is the most polar TS 1species possessing the largest dipole moment.It seems that the dipole moment comes into play especially through the bulk polarity and the NH–S hydrogen bonding effects as described in Fig.3.Consequently,environmental effects exert their influence on the TS 1species in the order of their dipole moments,such that 2TS 1B ,possessing the largest dipole moment,is stabilized more than all the other species,followed by 2TS 1A .The HS species,4TS 1B and 4TS 1A having smaller dipole moments,are stabilized less than the LS species.It is expected,therefore,that the bulk polarity and hydrogen bonding capability of the protein pocket can exert a remarkable effect on the regioselectivity of the N -dealkylation reaction of 3and cause a preference for N -demethylation over N -decyclopropylation,owing to the polar character of the TS 1species caused by the electron delocalization effect of the unique p Ph -p C–N conjugated system in 3.We also calculated the KIEs of the model reactions.The semiclassical values for the LS states are 5.1/4.5,and the Wigner-corrected values of 5.9/5.0indicate that tunneling effects are negligible on the LS states.However,the semiclassical KIEs on the HS state are 6.1/6.3,and Wigner-corrected KIEs of 8.6/8.8are much higher than the semiclassical values,indicating that tunneling effects are important for the HS states.These values are larger than the KIEs of a series of N -alky-N -cyclopropyl-p -chloroanilines studied in experiments 25–28probably because of the different N -substituents and the p -chloro of the benzene ring,but they are in line with the KIEs (ca.4–12)observed for aliphatic hydroxylation 64–67and O -dealkylation,68,69and are large enough to be consistent with a HAT mechanism for the loss of the cyclopropyl and the methyl group.Decomposition of carbinolanilineA carbinolaniline–heme complex is formed at the end of C a –H hydroxylation on the N -substituent.The second step of Path A (Path B)is the carbinolaniline decomposition to yield N -methylaniline (N -cyclopropylaniline)and cyclo-propanone (formaldehyde).Fig.5shows the energy profiles for the carbinolaniline decomposition process in the enzymatic en-vironment.The activation energies are about 35.5/30.5kcal/mol (36.3/31.8kcal/mol)on HS/LS states when environmental effects and ZPE corrections are taken into account.There is no significant spin density change through the reactant complex,transition state and product complex,thus this process is a proton-transfer one.From the discussion mentioned above we have seen that the C a –H hydroxylation step of Path A (Path B)is an exothermal process,and the carbinolaniline would decompose easily with this excess energy in the gas phase.However,in the enzymatic environment,this large excess energy is distributed to the protein pocket so fast that there is no or,at least,not sufficient energy left for the carbinolaniline to pass the high barrier of the decomposition process.Furthermore,carbinolaniline can escape from the heme pocket and be released to the nonenzymatic environment easily because of its low binding energy to the heme (HS:2.9kcal/mol,LS:6.4kcal/mol in Path A;HS:1.3kcal/mol,LS:7.3kcal/mol in Path B).Thus,decomposition of carbinolaniline will be performed in the nonenzymatic environment,consistent with the conventional concept.13Fig.5Energy profiles for the decomposition of carbinolaniline complex in the enzymatic environment.Values outside the parentheses are relative potential energies in the gas phase,while the values in parentheses involve corrections due to bulk polarity,NH–S hydrogen bonding,and ZPE.RC:reactant complex;TS:transition state;PC:product complex.All values are in kcal/mol relative to the reactant 4RC 1A .As the second step of the N -dealkylation,decomposition of the carbinolaniline requires a proton transfer from the hydroxyl oxygen to the aniline nitrogen,while the C–N bond between the alpha carbon and aniline nitrogen gradually breaks.We examined two different processes for the second step:one associated with a direct proton-transfer,and the other associated with a water-assisted proton-transfer.For the direct proton-transfer processD o w n l o a d e d b y D A L I A N U N I VE R S I T Y OF T E C H N O L OG Y o n 31 J u l y 2010P u b l i s h e d o n 19 N o v e m b e r 2008 o n h t t p ://p u b s .r s c .o r g | d o i :10.1039/B 810767Jinvolving transition state TS 2A (TS 2B ),the proton is transferred directly from the hydroxyl oxygen to the aniline nitrogen.For the water-assisted proton-transfer process involving transition state TS ¢2A (TS ¢2B ),the water molecule hydrogen-bonding with the aniline nitrogen gradually transfers a proton to the aniline nitrogen through the hydrogen bond,while the hydroxyl proton gradually transfers to the water oxygen.We can see from Fig.6that the activation energy of direct proton-transfer is 31.5kcal/mol in Path A (39.6kcal/mol in Path B)when the bulk solvation effect and ZPE correction are taken into account,whereas it decreases to 14.2kcal/mol (14.3kcal/mol in Path B)when this process is assisted by a molecule of water.It follows that the reaction process involving water-assisted proton transfer should dominate the carbinolaniline decomposition in the nonenzymatic environ-ment.Similar results were also reported for N -demethylation of N,N -dimethylanilines.45The large barrier decrease of the water-assisted proton-transfer process can be derived from the fact that decomposition of carbinolaniline is a pericyclic reaction,as is depicted in Fig.7.The transition state of the direct proton-transfer process is a rhomboid,whereas it becomes a hexagon after adding the water molecule.The smaller tension of the hexagon compared to that of the rhomboid leads to the decrease of thebarrier.Fig.6Energy profiles for nonenzymatic decomposition of carbino-lamine:(i),(iii)direct proton-transfer;(ii),(iv)water-assisted proton-trans-fer.Values outside the parentheses are relative potential energies,while the values in parentheses involve corrections due to bulk solvation and ZPE.RC:reactant complex;TS:transition state;PC:product complex.At the end of N -decyclopropylation,we can see from Fig.6a that the cyclopropyl ring lost during the reaction is not open,forming a ring-intact cyclopropanone (in PC ¢2of Fig.6a),and it will readily form the gem-diol hydrate of cyclopropanone (i.e.,cyclopropanone hydrate)that has been detected experimentally.23This further suggests that N -decyclopropylation of 3,unlike secondary amines,will not inactivate P450,which agrees precisely with experimental observations.23Fig.7Optimized structures for transitions states for the nonenzymatic decomposition of carbinolaniline:(i),(iii)direct proton-transfer;(ii),(iv)water-assisted proton-transfer.ConclusionsP450-catalyzed N -dealkylation of 3involves two steps,i.e.,C a –H hydroxylation on the N -substituent to form a carbinolaniline and decomposition of the carbinolaniline.The C a –H hydroxylation step of the reaction proceeds predominantly via the LS state in the SSM mechanism.The rate-limiting C a –H activation is an isotope-sensitive HAT step.With the lower barrier possessed by Path B,we predict a preference for N -demethylation over N -decyclopropylation,which is in accord with experimental studies but does not follow the normal D E-BDE correlation.Insight into the origin of the regioselectivity reveals that there is a unique p Ph -p C–N conjugated system in 3which cannot be found in normal hydrocarbons.The electron delocalization effect of the this p Ph -p C–N conjugated system makes the transition state possess a polar character,and regioselectivity of N -dealkylation of 3switches from a competition between N -decyclopropylation and N -demethylation to a clear preference for N -demethylation under the effect of the bulk polarity and hydrogen bonding capability in the protein pocket.Decomposition of carbinolaniline is a nonen-zymatic water-assisted proton-transfer process.The identification of cyclopropanone at the end of the N -decyclopropylation,which means intactness of the cyclopropyl group,further suggests that no enzyme inactivation will occur during the N -decyclopropylation of 3.References1P .R.Ortiz de Montellano,Cytochrome P450:Structure,Mechanism and Biochemistry ,Plenum,New Y ork,2nd edn,1995.2F .P .Guengerich,J.Biol.Chem.,1991,266,10019–10022.3F .P .Guengerich,Chem.Res.Toxicol.,2001,14,611–650.4M.J.Coon,Annu.Rev.Pharmacol.Toxicol.,2005,45,1–25.5F .P .Guengerich and T.L.Macdonald,FASEB J.,1990,4,2453–2459.6T.D.Porter and M.J.Coon,J.Biol.Chem.,1991,266,13469–13472.D o w n l o a d e d b y D A L I A N U N I VE R S I T Y OF T E C H N O L OG Y o n 31 J u l y 2010P u b l i s h e d o n 19 N o v e m b e r 2008 o n h t t p ://p u b s .r s c .o r g | d o i :10.1039/B 810767J。