Alkoxysilylated Derivatives of Double Four Ring Silicate
莫博赛替尼化学结构
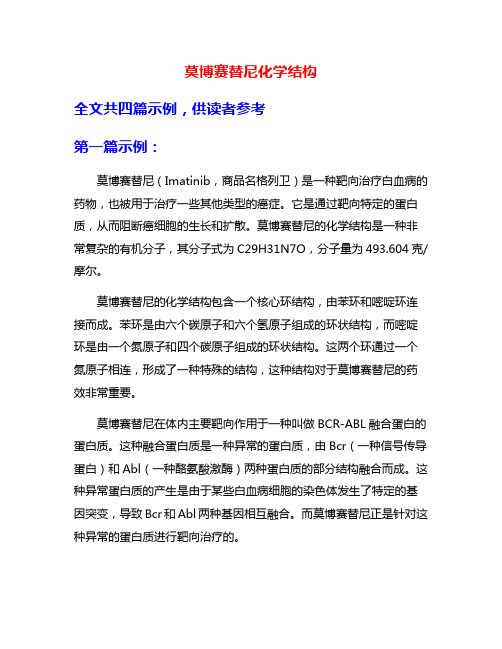
莫博赛替尼化学结构全文共四篇示例,供读者参考第一篇示例:莫博赛替尼(Imatinib,商品名格列卫)是一种靶向治疗白血病的药物,也被用于治疗一些其他类型的癌症。
它是通过靶向特定的蛋白质,从而阻断癌细胞的生长和扩散。
莫博赛替尼的化学结构是一种非常复杂的有机分子,其分子式为C29H31N7O,分子量为493.604克/摩尔。
莫博赛替尼的化学结构包含一个核心环结构,由苯环和嘧啶环连接而成。
苯环是由六个碳原子和六个氢原子组成的环状结构,而嘧啶环是由一个氮原子和四个碳原子组成的环状结构。
这两个环通过一个氮原子相连,形成了一种特殊的结构,这种结构对于莫博赛替尼的药效非常重要。
莫博赛替尼在体内主要靶向作用于一种叫做BCR-ABL融合蛋白的蛋白质。
这种融合蛋白质是一种异常的蛋白质,由Bcr(一种信号传导蛋白)和Abl(一种酪氨酸激酶)两种蛋白质的部分结构融合而成。
这种异常蛋白质的产生是由于某些白血病细胞的染色体发生了特定的基因突变,导致Bcr和Abl两种基因相互融合。
而莫博赛替尼正是针对这种异常的蛋白质进行靶向治疗的。
莫博赛替尼的化学结构设计和药效机制是经过长期的研究和开发的。
科学家们通过不断的实验和研究,逐渐了解了白血病细胞的基因突变以及融合蛋白质的结构和功能特点。
通过对这些信息的深入研究,他们最终设计出了莫博赛替尼这种能够精准干预癌细胞生长的药物。
莫博赛替尼在临床应用中被证实具有显著的疗效,尤其是对于慢性髓细胞白血病(CML)和胃肠间质瘤(GIST)等罕见白血病和恶性肿瘤的治疗效果非常显著。
通过连续服用莫博赛替尼,患者的白血细胞数量可以得到控制,进而延缓疾病的进展并提高生存率。
莫博赛替尼的治疗效果和安全性已经得到了许多临床试验和长期随访的验证,被广泛应用于临床治疗。
莫博赛替尼是一种非常重要的抗癌药物,通过靶向作用于异常蛋白质来抑制癌细胞的生长和扩散。
其化学结构复杂且精确,对于白血病和其他类型的癌症具有显著的治疗效果。
3例注射用奥沙利铂所致神经毒性不良反应分析及应对建议
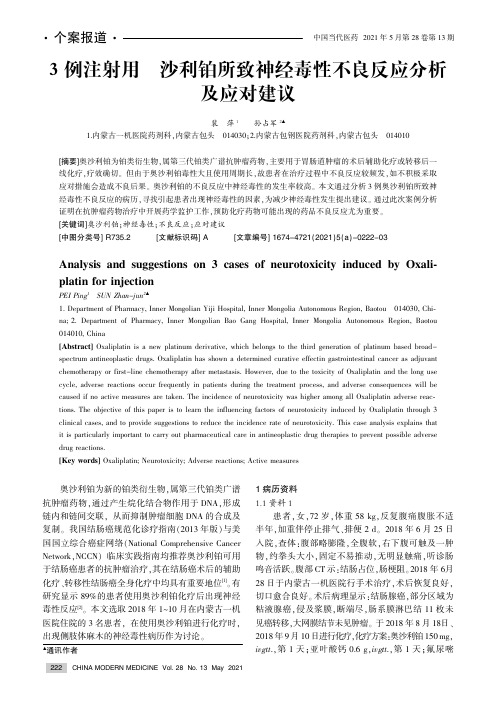
·个案报道·中国当代医药2021年5月第28卷第13期CHINA MODERN MEDICINE Vol.28No.13May 2021奥沙利铂为新的铂类衍生物,属第三代铂类广谱抗肿瘤药物,通过产生烷化结合物作用于DNA,形成链内和链间交联,从而抑制肿瘤细胞DNA 的合成及复制。
我国结肠癌规范化诊疗指南(2013年版)与美国国立综合癌症网络(National Comprehensive Cancer Network,NCCN)临床实践指南均推荐奥沙利铂可用于结肠癌患者的抗肿瘤治疗,其在结肠癌术后的辅助化疗、转移性结肠癌全身化疗中均具有重要地位[1]。
有研究显示89%的患者使用奥沙利铂化疗后出现神经毒性反应[2]。
本文选取2018年1~10月在内蒙古一机医院住院的3名患者,在使用奥沙利铂进行化疗时,出现侧肢体麻木的神经毒性病历作为讨论。
1病历资料1.1资料1患者,女,72岁,体重58kg,反复腹痛腹胀不适半年,加重伴停止排气、排便2d。
2018年6月25日入院,查体:腹部略膨隆,全腹软,右下腹可触及一肿物,约拳头大小,固定不易推动,无明显触痛,听诊肠鸣音活跃。
腹部CT 示:结肠占位,肠梗阻。
2018年6月28日于内蒙古一机医院行手术治疗,术后恢复良好,切口愈合良好。
术后病理显示:结肠腺癌,部分区域为粘液腺癌,侵及浆膜,断端尽,肠系膜淋巴结11枚未见癌转移,大网膜结节未见肿瘤。
于2018年8月18日、2018年9月10日进行化疗,化疗方案:奥沙利铂150mg,ivgtt .,第1天;亚叶酸钙0.6g,ivgtt .,第1天;氟尿嘧3例注射用奥沙利铂所致神经毒性不良反应分析及应对建议裴萍1孙占军2▲1.内蒙古一机医院药剂科,内蒙古包头014030;2.内蒙古包钢医院药剂科,内蒙古包头014010[摘要]奥沙利铂为铂类衍生物,属第三代铂类广谱抗肿瘤药物,主要用于胃肠道肿瘤的术后辅助化疗或转移后一线化疗,疗效确切。
甘草次酸衍生物修饰去甲斑蝥素脂质体在小鼠体内肝靶向性研究
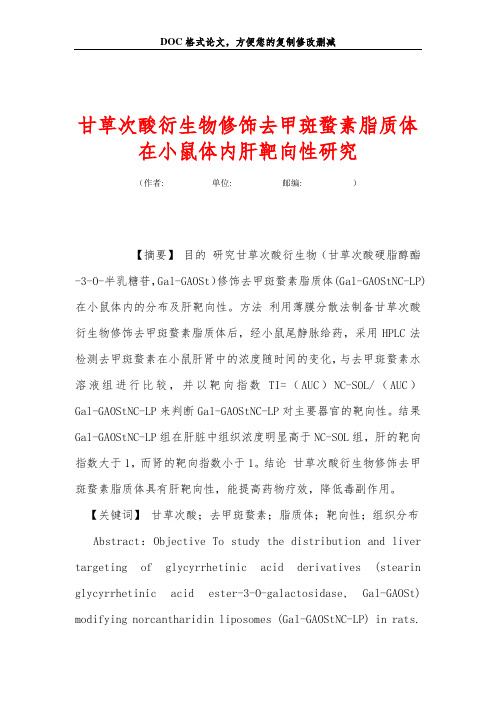
甘草次酸衍生物修饰去甲斑蝥素脂质体在小鼠体内肝靶向性研究(作者:___________单位: ___________邮编: ___________)【摘要】目的研究甘草次酸衍生物(甘草次酸硬脂醇酯-3-O-半乳糖苷,Gal-GAOSt)修饰去甲斑蝥素脂质体(Gal-GAOStNC-LP)在小鼠体内的分布及肝靶向性。
方法利用薄膜分散法制备甘草次酸衍生物修饰去甲斑蝥素脂质体后,经小鼠尾静脉给药,采用HPLC法检测去甲斑蝥素在小鼠肝肾中的浓度随时间的变化,与去甲斑蝥素水溶液组进行比较,并以靶向指数TI=(AUC)NC-SOL/(AUC)Gal-GAOStNC-LP来判断Gal-GAOStNC-LP对主要器官的靶向性。
结果Gal-GAOStNC-LP组在肝脏中组织浓度明显高于NC-SOL组,肝的靶向指数大于1,而肾的靶向指数小于1。
结论甘草次酸衍生物修饰去甲斑蝥素脂质体具有肝靶向性,能提高药物疗效,降低毒副作用。
【关键词】甘草次酸;去甲斑蝥素;脂质体;靶向性;组织分布 Abstract:Objective To study the distribution and liver targeting of glycyrrhetinic acid derivatives (stearin glycyrrhetinic acidester-3-O-galactosidase, Gal-GAOSt) modifying norcantharidin liposomes (Gal-GAOStNC-LP) in rats. Methods Making use of film decentralization to prepare glycyrrhetinic acid derivatives modifying norcantharidin liposomes, and then injecting it to the tail vein of rats. Making use of HPLC to detect the change of norcantharidin concentration in the rats’liver and kidney over time and compared it with norcantharidin solution group and targeting index TI = (AUC) NC-SOL / (AUC) Gal-GAOStNC-LP to determine the targeting of Gal-GAOStNC-LP to the main organs. Results Organization concentration of Gal-GAOStNC-LP group in the liver was significantly higher than NC-SOL group, the liver targeting index was greater than 1, and the targeting index of the kidney was less than 1. Conclusions Glycyrrhetinic acid derivatives modifying norcantharidin liposomes possess liver targeting that can improve the efficacy of drugs, reduce toxicity and side effect.Key words:glycyrrheticacid;norcantharidin;liposome;targeting;distributio n去甲斑蝥素具有抗癌,保护肝细胞和调节免疫等作用[1],可用于肝癌等恶性肿瘤的治疗,是一种具有潜力的抗肿瘤中药,但是其毒性很大容易对机体产生不良反应。
恩曲替尼化学式-概述说明以及解释
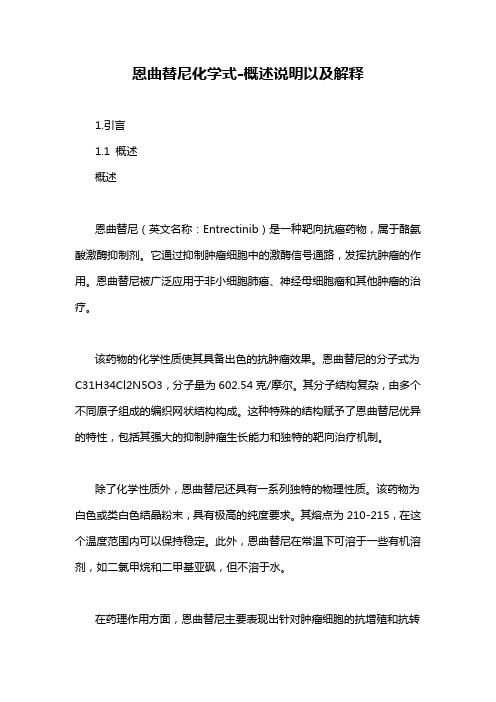
恩曲替尼化学式-概述说明以及解释1.引言1.1 概述概述恩曲替尼(英文名称:Entrectinib)是一种靶向抗癌药物,属于酪氨酸激酶抑制剂。
它通过抑制肿瘤细胞中的激酶信号通路,发挥抗肿瘤的作用。
恩曲替尼被广泛应用于非小细胞肺癌、神经母细胞瘤和其他肿瘤的治疗。
该药物的化学性质使其具备出色的抗肿瘤效果。
恩曲替尼的分子式为C31H34Cl2N5O3,分子量为602.54克/摩尔。
其分子结构复杂,由多个不同原子组成的编织网状结构构成。
这种特殊的结构赋予了恩曲替尼优异的特性,包括其强大的抑制肿瘤生长能力和独特的靶向治疗机制。
除了化学性质外,恩曲替尼还具有一系列独特的物理性质。
该药物为白色或类白色结晶粉末,具有极高的纯度要求。
其熔点为210-215,在这个温度范围内可以保持稳定。
此外,恩曲替尼在常温下可溶于一些有机溶剂,如二氯甲烷和二甲基亚砜,但不溶于水。
在药理作用方面,恩曲替尼主要表现出针对肿瘤细胞的抗增殖和抗转移能力。
它通过干扰肿瘤细胞的激酶信号通路,阻止肿瘤细胞的分裂和生长。
此外,恩曲替尼还具有特异性靶向治疗作用,能够选择性地抑制特定的激酶,从而实现精确的治疗效果。
然而,恩曲替尼也存在一些副作用,如恶心、呕吐、疲劳和食欲不振等,这些副作用需在使用时留意并及时处理。
综上所述,恩曲替尼作为一种靶向抗肿瘤药物,具有复杂的化学性质、独特的物理性质以及较广泛的药理作用。
进一步的研究和应用将有助于更好地发掘恩曲替尼的潜力,为肿瘤治疗提供新的突破和可能性。
1.2 文章结构文章结构部分的内容如下:文章结构部分主要介绍了整篇文章的组织结构和内容安排。
本文的目录分为引言、正文和结论三个部分。
引言部分主要是对整篇文章的背景和目的进行概述,并对恩曲替尼的化学式进行引入。
接着,文章结构部分将详细介绍恩曲替尼的化学性质、物理性质和药理作用。
最后,结论部分将对恩曲替尼的化学性质、物理性质和药理作用进行总结。
在正文部分,恩曲替尼的化学性质将包括分子式、分子量和结构式的介绍。
反溶剂诱导结晶法英文
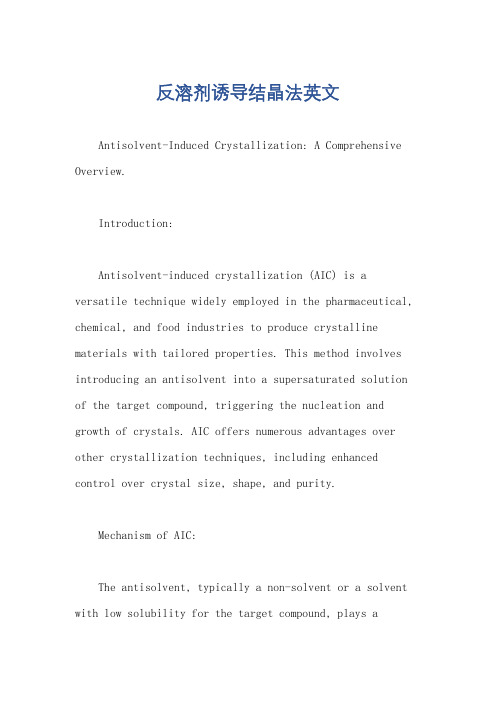
反溶剂诱导结晶法英文Antisolvent-Induced Crystallization: A Comprehensive Overview.Introduction:Antisolvent-induced crystallization (AIC) is a versatile technique widely employed in the pharmaceutical, chemical, and food industries to produce crystalline materials with tailored properties. This method involves introducing an antisolvent into a supersaturated solution of the target compound, triggering the nucleation and growth of crystals. AIC offers numerous advantages over other crystallization techniques, including enhanced control over crystal size, shape, and purity.Mechanism of AIC:The antisolvent, typically a non-solvent or a solvent with low solubility for the target compound, plays acrucial role in the AIC process. When added to a supersaturated solution, the antisolvent reduces the solubility of the solute, leading to the formation of a metastable zone. Within this zone, small crystal nuclei form and begin to grow. The antisolvent concentration, temperature, and solution composition influence the nucleation and growth kinetics, ultimately determining the characteristics of the final crystals.Advantages of AIC:AIC offers several advantages over conventional crystallization methods, including:Control over Crystal Morphology: AIC allows for the manipulation of crystal size, shape, and surface structure by varying process parameters such as antisolvent type, concentration, and temperature.Enhanced Purity: The antisolvent acts as a washing agent, removing impurities from the growing crystals and improving their purity.Scalability: AIC is a scalable process suitable for both small-scale laboratory experiments and large-scale industrial production.Energy Efficiency: Compared to other crystallization techniques, AIC often requires lower energy input due to reduced evaporation and milder operating conditions.Applications of AIC:AIC finds applications in a wide range of industries, including:Pharmaceuticals:Production of active pharmaceutical ingredients (APIs) with controlled bioavailability and dissolution rates.Development of drug delivery systems with specific release profiles.Chemicals:Synthesis of fine chemicals and specialty materials.Crystallization of inorganic compounds for electronic and optical applications.Food:Production of food additives and flavors.Crystallization of sugars and sweeteners.Process Parameters:The success of AIC depends on the careful optimization of several process parameters, including:Antisolvent Selection: The choice of antisolvent is crucial and depends on its solubility characteristics, miscibility with the solvent, and ability to promote nucleation.Antisolvent Concentration: The concentration of the antisolvent determines the degree of supersaturation andthe nucleation rate.Temperature: Temperature plays a significant role in crystal growth and morphology. Lower temperatures generally favor smaller crystal sizes.Mixing: Efficient mixing is essential for uniform distribution of the antisolvent and to preventagglomeration of crystals.Crystal Seeding: Seeding with pre-formed crystals can control nucleation and promote the growth of specificcrystal faces.Equipment for AIC:AIC can be carried out using various types of equipment, such as:Batch Crystallizers: Simple vessels where the antisolvent is added to a supersaturated solution.Continuous Crystallizers: Allow for continuous operation and better control over crystal growth.Fluidized Bed Crystallizers: Suspend crystals in a fluidized bed, facilitating efficient mass transfer and crystal growth.Challenges and Considerations:Despite its versatility, AIC also faces some challenges:Crystal Agglomeration: High supersaturation or insufficient mixing can lead to agglomeration, resulting in non-uniform crystal properties.Nucleation Control: Controlling the nucleation rate is crucial to obtain the desired crystal size and distribution.Solvent Selection: The choice of solvent andantisolvent combination must consider their solubility and stability under process conditions.Conclusion:Antisolvent-induced crystallization is a powerful technique that offers significant advantages for the production of crystalline materials with tailored properties. Its versatility, scalability, and ability to control crystal morphology and purity make AIC a valuable tool for various industries, including pharmaceuticals, chemicals, and food. Continued research and development in AIC aim to further optimize the process and expand its applications.。
化妆品原料中英文对照 a到z
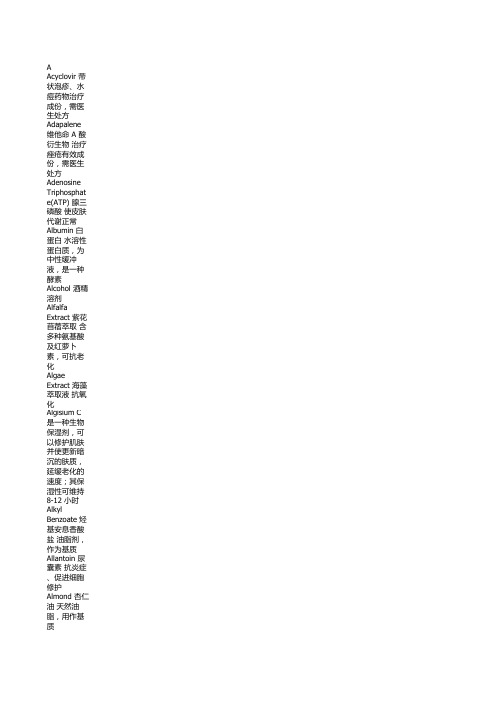
Burdock 牛 蒡 消毒、预 防粉刺、促 进细胞生长 、抗发炎
Butyl Methoxydibe nzoylmethan e 化学性防 晒成分,属 Parsol 1789 类,罕见过 敏反应 Butyl Paraben 丁 酯 防腐剂 Butyl Stelene Glycol 丁二 醇 保湿 Butylhydrox yanisol 羟基 茴香二丁酯 酸化防止剂
Calendula Extract 金盏 花萃取 具舒 缓、安抚敏 感肌肤等功 效 Camellia
Sinensis Extract 山茶 萃取,茶多 酚 抗氧化 Camphor 樟 树 抗痒、防 过敏 Candelilla Wax 墈地里 拉蜡 浓度增 强剂 Caprylic/Cap
ric
Triglyceride 三甘油酯 皮 肤润滑剂 Carbomer 高 份子胶 浓度 增强剂 Carbopol 羧 乙烯聚合物 赋型剂 Carboxymet hyl Chitin 几 丁质衍生物 来自虾蟹外 壳,为一高 分子量之粘 多醣体,具 有保湿作用 Carnauba Wax 棕榈蜡 增加光泽感 Carrageenan 鹿角菜胶 保 湿 Carrot Oil 胡 萝卜油 可促 使伤口愈合 、镇痛、滋 养、消毒、 抗老化 Carthamus
Glucoside(A AG) 维他命 C 甘醣 维他 命 C 衍生 物,为卫生 署公布有效 美白成份之 一 Ascorbyl Pamitate 维 他命 C 棕榈 酸盐 一种脂 溶性维他命 C ,是安定 的维他命 C Ascorbyl Stearate 酯 化 C 安定的 维他命 C Ascorbyl
Amniotic Fluid 羊膜液 含丰富肌肤 所需的胺基 酸 Angelica
Sinensis
木质纤维素制备寡聚糖及其在绿色制革中的应用
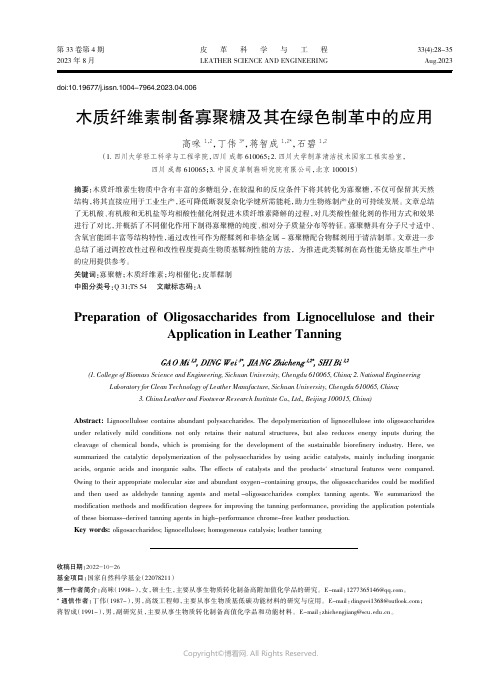
doi:10.19677/j.issn.1004-7964.2023.04.006木质纤维素制备寡聚糖及其在绿色制革中的应用高咪1,2,丁伟3*,蒋智成1,2*,石碧1,2(1.四川大学轻工科学与工程学院,四川成都610065;2.四川大学制革清洁技术国家工程实验室,四川成都610065;3.中国皮革制鞋研究院有限公司,北京100015)摘要:木质纤维素生物质中含有丰富的多糖组分,在较温和的反应条件下将其转化为寡聚糖,不仅可保留其天然结构,将其直接应用于工业生产,还可降低断裂复杂化学键所需能耗,助力生物炼制产业的可持续发展。
文章总结了无机酸、有机酸和无机盐等均相酸性催化剂促进木质纤维素降解的过程,对几类酸性催化剂的作用方式和效果进行了对比,并概括了不同催化作用下制得寡聚糖的纯度、相对分子质量分布等特征。
寡聚糖具有分子尺寸适中、含氧官能团丰富等结构特性,通过改性可作为醛鞣剂和非铬金属-寡聚糖配合物鞣剂用于清洁制革。
文章进一步总结了通过调控改性过程和改性程度提高生物质基鞣剂性能的方法,为推进此类鞣剂在高性能无铬皮革生产中的应用提供参考。
关键词:寡聚糖;木质纤维素;均相催化;皮革鞣制中图分类号:Q31;TS54文献标志码:APreparation of Oligosaccharides from Lignocellulose and theirApplication in Leather Tanning(1.College of Biomass Science and Engineering,Sichuan University,Chengdu610065,China;2.National EngineeringLaboratory for Clean Technology of Leather Manufacture,Sichuan University,Chengdu610065,China;3.China Leather and Footwear Research Institute Co.,Ltd.,Beijing100015,China)Abstract:Lignocellulose contains abundant polysaccharides.The depolymerization of lignocellulose into oligosaccharides under relatively mild conditions not only retains their natural structures,but also reduces energy inputs during the cleavage of chemical bonds,which is promising for the development of the sustainable biorefinery industry.Here,we summarized the catalytic depolymerization of the polysaccharides by using acidic catalysts,mainly including inorganic acids,organic acids and inorganic salts.The effects of catalysts and the products'structural features were compared. Owing to their appropriate molecular size and abundant oxygen-containing groups,the oligosaccharides could be modified and then used as aldehyde tanning agents and metal-oligosaccharides complex tanning agents.We summarized the modification methods and modification degrees for improving the tanning performance,providing the application potentials of these biomass-derived tanning agents in high-performance chrome-free leather production.Key words:oligosaccharides;lignocellulose;homogeneous catalysis;leather tanning收稿日期:2022-10-26基金项目:国家自然科学基金(22078211)第一作者简介:高咪(1998-),女,硕士生,主要从事生物质转化制备高附加值化学品的研究。
二氧化钛表面超强酸化光氧复合降解罗丹明B

第40卷第2期2021年3月Vol.40No.2Mar.2021大连工业大学学报JournalofDalianPolytechnicUniversityDOI:10.19670/ki.dlgydxxb.2021.0210二氧化钛表面超强酸化光氧复合降解罗丹明B温宇,杨大伟(大连工业大学轻工与化学工程学院,辽宁大连116034)摘要:采用共结晶方法制备了锌锆共掺杂的介孔二氧化钛,前驱体用硫酸处理使其具有超强酸性。
将制备的介孔二氧化钛用于降解废水模拟物罗丹明B,测试其光催化与氧催化降解能力。
通过紫外-可见分光光度计、X射线衍射、电镜扫描等对催化剂进行表征,实验结果表明,在强酸修饰二氧化钛前驱体的影响下,掺杂锌锆的介孔二氧化钛具有光催化与氧催化活性。
锌锆共掺杂介孔二氧化钛的光催化与氧催化效率分别达到了72%与25%o硫酸处理后在TiO2与掺杂原子表明形成酸性中心,在无光条件下氧化降解废水效率为30%,提高了降解效率。
关键词:二氧化钛;光催化;酸催化;罗丹明B中图分类号:X703.5文献标志码:A文章编号:1674-1404(2021)02-0136-04Composite degradation of rhodamine B using TiO2withphotocatalytic oxygen and super acidWEN Yu,YANG Dawei(SchoolofLightndustryandChemicalEngineering,DalianPolytechnicUniversity,Dalian116034,China) Abstract:The mesoporous titania doped with zinc oxide,zirconium dioxide,zinc and zirconium were prepared by the co-crystallization method and the precursor of mesoporous titania was pretreated with sulfuric acid to endowed it super acidic.The mesoporous titania was used for degradation of rhodamine B in simulated wastewater and its photocatalytic activity and oxygen catalytic ability was analyzed by UV-visible spectrophotometer,X ray diffraction,scanning electron microscopy.The results showed that the T1O2doped metal oxides and super acid exhibited excellent photocatalytic and oxygen catalytic ability.The degradation rate of rhodamine B photocatalyzed and oxygen catalyzed by the prepared catalysts were72%and25%,respectively.After treatment with sulfuric acid,the acidic centers were formed between the doped atoms and the surface of titanium dioxide,which improved the oxygen degrading efficiency of wastewater to30%.Keywords:TiO2;photocatalytic;acidic catalysis;rhodamine B0引言工业生产中生成的有机废水对环境造成严重污染,国家对废水排放标准执行越来越严格,如何降低或消除有机废水中大分子有机物成为研究的重点。
双草酸硼酸锂libob是一种新型的锂盐具有很
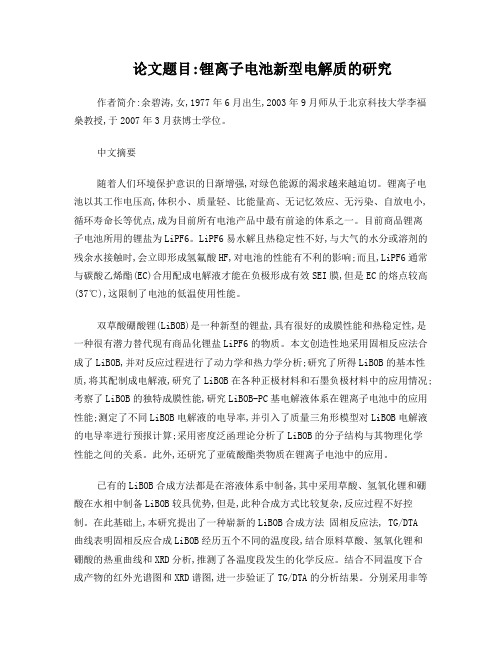
论文题目:锂离子电池新型电解质的研究作者简介:余碧涛,女,1977年6月出生,2003年9月师从于北京科技大学李福燊教授,于2007年3月获博士学位。
中文摘要随着人们环境保护意识的日渐增强,对绿色能源的渴求越来越迫切。
锂离子电池以其工作电压高,体积小、质量轻、比能量高、无记忆效应、无污染、自放电小,循环寿命长等优点,成为目前所有电池产品中最有前途的体系之一。
目前商品锂离子电池所用的锂盐为LiPF6。
LiPF6易水解且热稳定性不好,与大气的水分或溶剂的残余水接触时,会立即形成氢氟酸HF,对电池的性能有不利的影响;而且,LiPF6通常与碳酸乙烯酯(EC)合用配成电解液才能在负极形成有效SEI膜,但是EC的熔点较高(37℃),这限制了电池的低温使用性能。
双草酸硼酸锂(LiBOB)是一种新型的锂盐,具有很好的成膜性能和热稳定性,是一种很有潜力替代现有商品化锂盐LiPF6的物质。
本文创造性地采用固相反应法合成了LiBOB,并对反应过程进行了动力学和热力学分析;研究了所得LiBOB的基本性质,将其配制成电解液,研究了LiBOB在各种正极材料和石墨负极材料中的应用情况;考察了LiBOB的独特成膜性能,研究LiBOB-PC基电解液体系在锂离子电池中的应用性能;测定了不同LiBOB电解液的电导率,并引入了质量三角形模型对LiBOB电解液的电导率进行预报计算;采用密度泛函理论分析了LiBOB的分子结构与其物理化学性能之间的关系。
此外,还研究了亚硫酸酯类物质在锂离子电池中的应用。
已有的LiBOB合成方法都是在溶液体系中制备,其中采用草酸、氢氧化锂和硼酸在水相中制备LiBOB较具优势,但是,此种合成方式比较复杂,反应过程不好控制。
在此基础上,本研究提出了一种崭新的LiBOB合成方法固相反应法, TG/DTA曲线表明固相反应合成LiBOB经历五个不同的温度段,结合原料草酸、氢氧化锂和硼酸的热重曲线和XRD分析,推测了各温度段发生的化学反应。
TW 46化学化工
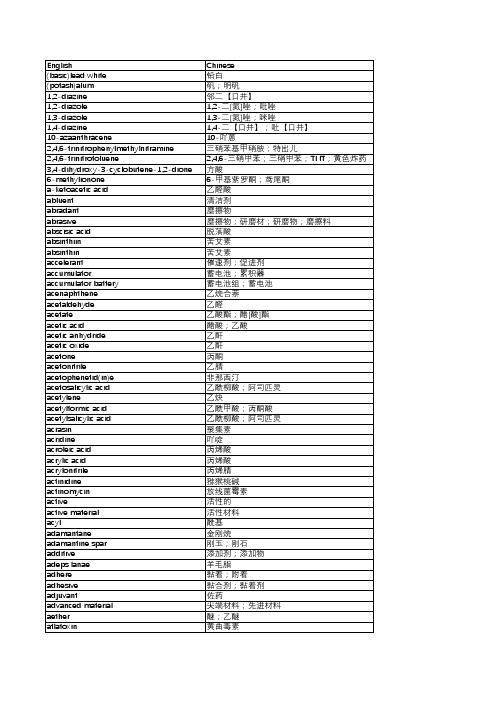
焙粉 焙用碱 巴巴斯可 {毒鱼藤} 巴比妥 钡熔剂 障壁层光电池 重子 介子 碱式碳酸铅 罗勒[精]油 篮烯 [刺]梧桐[树]胶;黄蓍胶 电池;电池组;电瓶 蜂蜡 苯甲醛 吲哚 苯胺;胺苯 苯 六氯化苯 苯甲酸;苄酸;安息[香]酸 苯甲酰磺酰亚胺;糖精 苯 苯 苯并芘 苯并吡啶 苯甲酰磺酰亚胺;糖精 盆苯 小蘗碱 香柑素 香柠檬素;佛手柑素 内盐;甜菜碱 黏结材料 生物沼气 铋华;砷铋矿 铋华 苦土 苦盐;泻盐 苦卤 井盐苦卤 黑琥珀 焦糖;闪锌矿;马列兰栎树 黑液 黑液 爆破药 漂白粉 漂白粉浓缩物 胆矾 胆蓝 蓝石;胆矾 熟[炼]油 骨泥 骨粉;细骨粉 骨胶 骨粉 骨油
borane borax Bordeaux mixture boric acid borneol bornyl alcohol boron carbide boron mud boson bouillie bordelaise brasque brick bridged hydrocarbon brine brucine burnt sugar bus-bar bus-barwire butadiene butanedioic acid buthotoxin by-product caffeine caffeine cage compound calamine calcitriol calcium acetylide calcium carbide calcium hydroxide calcium pantothenate calomel calp calxchlorinate camouflage material camphene camphor camptothecin capsaicin capsaicine capsanthin capsicine caramel carane carbamamidine carbamide carbazole carbene carbide carbinol carbofos carbohydrate(s) carbohydrate(s) carboline carbon dioxide
用于治疗癌症的萘二甲酰亚胺衍生物[发明专利]
![用于治疗癌症的萘二甲酰亚胺衍生物[发明专利]](https://img.taocdn.com/s3/m/17f30daccf84b9d529ea7ad6.png)
专利名称:用于治疗癌症的萘二甲酰亚胺衍生物
专利类型:发明专利
发明人:E·范夸克比克,G·西蒙,L·范登霍夫,R·基斯,F·达罗申请号:CN200580013866.5
申请日:20050504
公开号:CN1950341A
公开日:
20070418
专利内容由知识产权出版社提供
摘要:使用新的通式(I)和(II)的取代的萘二甲酰亚胺衍生物,其药学上可接受的盐及其溶剂化物来制造药物组合物,用于治疗细胞增殖疾病如癌症。
本发明还提供制造这些衍生物的方法。
申请人:优尼拜尔斯金股份有限公司
地址:比利时布鲁塞尔
国籍:BE
代理机构:上海专利商标事务所有限公司
代理人:沙永生
更多信息请下载全文后查看。
《胡椒酸钾对黑色素瘤细胞的影响及其与Avasimibe联用的抑癌效果》范文
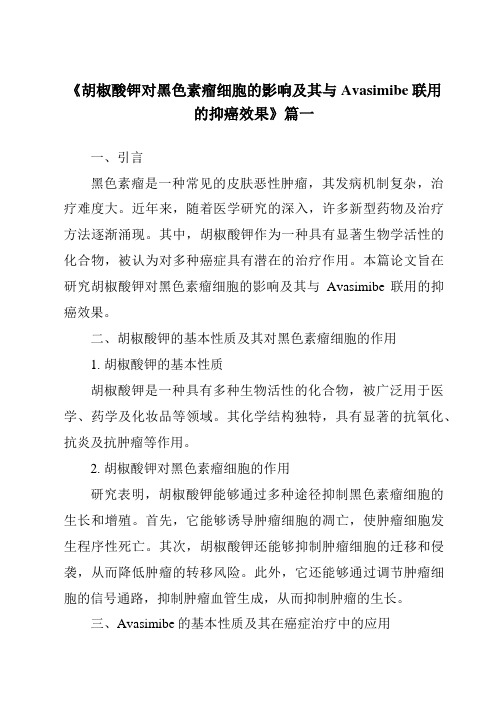
《胡椒酸钾对黑色素瘤细胞的影响及其与Avasimibe联用的抑癌效果》篇一一、引言黑色素瘤是一种常见的皮肤恶性肿瘤,其发病机制复杂,治疗难度大。
近年来,随着医学研究的深入,许多新型药物及治疗方法逐渐涌现。
其中,胡椒酸钾作为一种具有显著生物学活性的化合物,被认为对多种癌症具有潜在的治疗作用。
本篇论文旨在研究胡椒酸钾对黑色素瘤细胞的影响及其与Avasimibe联用的抑癌效果。
二、胡椒酸钾的基本性质及其对黑色素瘤细胞的作用1. 胡椒酸钾的基本性质胡椒酸钾是一种具有多种生物活性的化合物,被广泛用于医学、药学及化妆品等领域。
其化学结构独特,具有显著的抗氧化、抗炎及抗肿瘤等作用。
2. 胡椒酸钾对黑色素瘤细胞的作用研究表明,胡椒酸钾能够通过多种途径抑制黑色素瘤细胞的生长和增殖。
首先,它能够诱导肿瘤细胞的凋亡,使肿瘤细胞发生程序性死亡。
其次,胡椒酸钾还能够抑制肿瘤细胞的迁移和侵袭,从而降低肿瘤的转移风险。
此外,它还能够通过调节肿瘤细胞的信号通路,抑制肿瘤血管生成,从而抑制肿瘤的生长。
三、Avasimibe的基本性质及其在癌症治疗中的应用Avasimibe是一种新型的胆固醇抑制剂,具有显著的抗癌作用。
它能够通过抑制胆固醇的合成,从而影响肿瘤细胞的生长和增殖。
同时,Avasimibe还能够与其他抗癌药物联合使用,增强其治疗效果。
四、胡椒酸钾与Avasimibe联用的抑癌效果将胡椒酸钾与Avasimibe联用后,两者的协同作用能够显著提高对黑色素瘤细胞的抑制效果。
一方面,Avasimibe通过抑制胆固醇的合成,降低肿瘤细胞的能量供应,从而增强其对抗药物的敏感性;另一方面,胡椒酸钾则通过诱导肿瘤细胞凋亡、抑制肿瘤细胞迁移和侵袭等途径,进一步增强抑癌效果。
此外,两者联用还能够调节肿瘤细胞的信号通路,从而影响肿瘤的生长和增殖。
五、实验结果与讨论通过实验数据表明,将胡椒酸钾与Avasimibe联用后,黑色素瘤细胞的生长受到明显抑制。
富勒烯衍生物
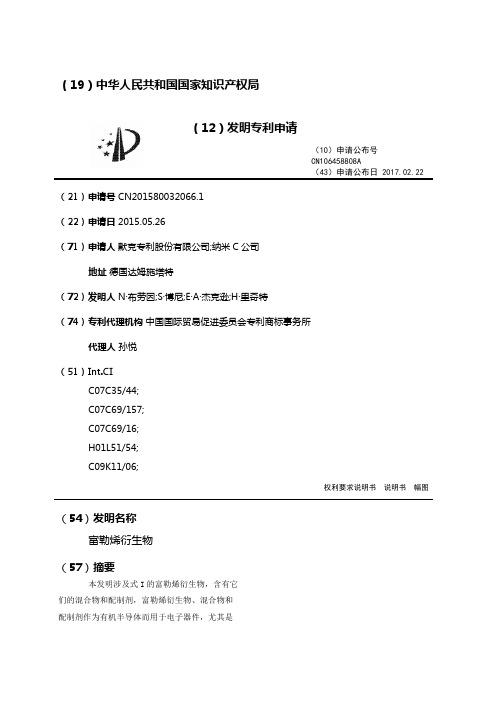
(19)中华人民共和国国家知识产权局
(12)发明专利申请
(10)申请公布号
CN106458808A
(43)申请公布日 2017.02.22(21)申请号CN201580032066.1
(22)申请日2015.05.26
(71)申请人默克专利股份有限公司;纳米C公司
地址德国达姆施塔特
(72)发明人N·布劳因;S·博尼;E·A·杰克逊;H·里奇特
(74)专利代理机构中国国际贸易促进委员会专利商标事务所
代理人孙悦
(51)Int.CI
C07C35/44;
C07C69/157;
C07C69/16;
H01L51/54;
C09K11/06;
权利要求说明书说明书幅图
(54)发明名称
富勒烯衍生物
(57)摘要
本发明涉及式I的富勒烯衍生物,含有它
们的混合物和配制剂,富勒烯衍生物、混合物和
配制剂作为有机半导体而用于电子器件,尤其是
有机光伏(OPV)器件和有机光电探测器(OPD)的用
途或用于制备电子器件,尤其是有机光伏(OPV)器
件和有机光电探测器(OPD)的用途,以及包含这些
富勒烯衍生物、混合物或配制剂或由这些富勒烯
衍生物、混合物或配制剂所制得的电子器件。
法律状态
法律状态公告日法律状态信息法律状态
2017-02-22公开公开
2017-02-22公开公开
2017-07-11实质审查的生效实质审查的生效
权利要求说明书富勒烯衍生物的权利要求说明书内容是....请下载后查看
说明书富勒烯衍生物的说明书内容是....请下载后查看。
- 1、下载文档前请自行甄别文档内容的完整性,平台不提供额外的编辑、内容补充、找答案等附加服务。
- 2、"仅部分预览"的文档,不可在线预览部分如存在完整性等问题,可反馈申请退款(可完整预览的文档不适用该条件!)。
- 3、如文档侵犯您的权益,请联系客服反馈,我们会尽快为您处理(人工客服工作时间:9:00-18:30)。
Alkoxysilylated-Derivatives of Double-Four-Ring Silicate as Novel Building Blocks of Silica-Based Materials†Yoshiaki Hagiwara,‡Atsushi Shimojima,*,§,|and Kazuyuki Kuroda*,‡,§,#Department of Applied Chemistry,Waseda University,Ohkubo-3,Shinjuku-ku,Tokyo169-8555,Japan,Kagami Memorial Laboratory for Materials Science and Technology,Waseda University,Nishiwaseda-2,Shinjuku-ku,Tokyo169-0051,Japan,and CREST,Japan Scienceand Technology Agency(JST),JapanReceived June18,2007.Revised Manuscript Received July11,2007Novel oligomeric alkoxysilanes consisting of double-four-ring(D4R)units functionalized with mono-,di-,and trialkoxysilyl groups(designated as1,2,and3,respectively)were designed as nanobuilding blocks for synthesizing silica-based nanomaterials.Hydrolysis and polycondensation of these oligomers were performed under acidic conditions.The gelation occurred much faster than monomeric,dimeric,and cubic octameric alkoxysilanes,which should depend on both molecular size and siloxane structures.Structural analyses of the xerogels derived from1-3strongly suggested that the D4R units are at least partly retained in their frameworks.The number of alkoxy groups had a large effect on their porosities: the xerogel derived from2was a microporous solid with the BET surface area of600m2 g-1,whereas1-and3-derived xerogels had very low BET surface areas.Furthermore, mesostructured silica films were successfully synthesized from2and3by using amphiphilic triblock copolymer surfactant as a structure-directing agent.Such an approach will lead to the construction of molecularly designed siloxane networks.IntroductionSol-gel chemistry of alkoxysilanes represents one of the fundamental approaches for synthesizing silica-based materials under mild conditions.1The structural control of silica at various length scales is important not only for basic research but for many practical applications including catalysis and adsorption.The use of oligosiloxane units with well-defined structures as molecular building blocks is promising for the rational design of siloxane structures having at least a short-range order.Moreover,the precise control over the as sembly of those building blocks is a significant issue, leading to the construction of hierarchically ordered materials.Siloxane cage compounds,such as those having a double-four-ring(D4R)unit,are particularly useful as molecular building blocks because of their rigid and symmetrical frameworks.2,3It is known that D4R sili-cate anions(Si8O208-)are formed in high yield in alkaline silicate solutions containing tetramethylam-monium(TMA)cations.4Alternatively,organic deriva-tives(R8Si8O12,where R)H or organic groups)can be obtained by controlled hydrolysis and polycondensation of the corresponding trifunctional silanes.5Cross-linking of D4R units via Si-O-Si linkages is expected to offer a building block approach to molecularly ordered silica like zeolites.6However,only a few attempts have been reported so far,whereas a variety of hybrid materials have been prepared by covalent linking of D4R units via Si-C and C-C bonds.7Klemperer and co-workers reported the preparation of microporous silica xerogels by the sol-gel processes of cubic octameric alkoxysilane, an alkoxy-derivative of the D4R silicate((MeO)8Si8O12).8 The mesoscopic organization of D4R silicate anions has also been achieved by electrostatic interaction with cationic surfactants,forming lamellar,2D hexagonal, and cubic structures.9The molecular design of even larger building blocks with alkoxy-functionality should lead to the structural and morphological diversity of resulting materials.An interesting approach is to functionalize D4R units with†Part of the“Templated Materials Special Issue”.*To whom correspondence should be addressed.Phone&Fax:81-3-5286-3199.E-mail:kuroda@waseda.jp(K.K.);shimoji@chemsys.t.u-tokyo.ac.jp(A.S.).‡Department of Applied Chemistry,Waseda University.§CREST,Japan Science and Technology Agency.||Present address:Department of Chemical System Engineering, The University of Tokyo,Hongo-7,Bunkyo-ku,Tokyo113-8656,Japan #Kagami Memorial Laboratory for Materials Science and Technol-ogy,Waseda University.(1)Brinker, C.J.;Scherer,G.W.Sol-Gel Science;Academic Press:San Diego,CA,1990.(2)Laine,R.M.J.Mater.Chem.2005,15,3725.(3)Harrison,anomet.Chem.1997,542,141.(4)Hoebbel,D.;Garzo´,G.;Engelhardt,G.;Vargha,A.Z.Anorg. Allg.Chem.1982,494,31.(5)(a)Agaskar,P.A.Inorg.Chem.1991,30,2707.(b)Bassindale,A.R.;Liu,Z.;MacKinnon,I.A.;Taylor,P.G.;Yang,Y.;Light,M.E.; Horton,P.N.;Hursthouse,M.B.Dalton Trans.2003,2945.(6)Morris,R.E.J.Mater.Chem.2005,15,931.(7)(a)Hoebbel,D.;Endres,K.;Reinert,T.;Pitsch,I.J.Non-Cryst. Solids1994,176,179.(b)Harrison,P.G.;Kannengiesser,R.Chem. Commun.1996,415.(c)Morrison,J.J.;Love,C.J.;Manson,B.W.; Shannon,I.J.;Morris,R.E.J.Mater.Chem.2002,12,3208.(d)Zhang, C.;Babonneau,F.;Bonhomme,C.;Laine,R.M.;Soles,C.L.;Hristov,H.A.;Yee,A.F.J.Am.Chem.Soc.1998,120,8380.(8)(a)Day,V.W.;Klemperer,W.G.;Mainz,V.V.;Millar,D.M.J. Am.Chem.Soc.1985,107,8262.(b)Klemperer,W.G.;Mainz,V.V.; Millar,D.M.Mater.Res.Soc.Symp.Proc.1986,73,3.(c)Cagle,P.C.;Klemperer,W.G.;Simmons,C.A.Mater.Res.Soc.Symp.Proc. 1990,180,29.10.1021/cm0716194CCC:$27.50©xxxx American Chemical SocietyPAGE EST:6.7Published on Web09/08/2007additional eight alkoxysilyl groups,leading to spherical molecules with 16silicon atoms.Most recently,octavi-nyl-substituted D4R was hydrosilylated with HSi(OEt)3to design novel building blocks that formed mesoporous silsesquioxane-based materials.10In contrast to this strategy,we focus our efforts on designing a new type of siloxane-based oligomers,where all of the Si atoms are connected via Si -O -Si linkages.Such molecules can be directly synthesized by silylation of the corner SiO -groups of D4R silicates.Silylated-derivatives containing functional groups such as Si -H and Si -CH d CH 2have been prepared by silylation of D4R silicates with organochlorosilanes.11Also,porous solids have been prepared by covalent linking of D4R units by silylation with dimethyldichlorosilanes.12However,to the best of our knowledge,functionalization of D4R units with alkoxysilyl groups has been unprecedented,although such molecules are potentially useful for the sol -gel synthesis of new materials.In this paper,we report the synthesis of novel alkoxysilylated-derivatives of D4R silicates and their use as building blocks for constructing silica-based nanomaterials.Under well-regulated conditions,we succeeded in synthesizing three types of oligomeric alkoxysilanes,designated as 1,2,and 3(Scheme 1),by silylation of D4R silicate with dimethylethoxychlorosi-lane [Me 2(EtO)SiCl],methyldiethoxychlorosilane [Me-(EtO)2SiCl],and triethoxychlorosilane [Si(EtO)3SiCl],respectively.The reaction relies on the much higher reactivity of Si -Cl groups than the Si -OEt groups;therefore,the Si -Cl groups preferentially react with Si -OH (or O -)groups of silicate species.13These oligo-mers consist of a rigid D4R core and branched alkox-ysilyl groups with different numbers of alkoxy groups,which should affect the structure of the final products.The hydrolysis and polycondensation behaviors of 1,2,and 3under acidic conditions were compared with those of monomeric,dimeric,and cubic octameric alkoxysi-lanes.14Detailed characterization of the resulting xe-rogels was performed to understand their structural features.Furthermore,amphiphilic triblock copolymersurfactant (EO 20PO 70EO 20)was used as a structure-directing agent to form thin films with hierarchical structures.Experimental SectionMaterials.Dimethyldichlorosilane,methyltrichlorosilane,tetrachlorosilane (Tokyo Kasei Co.,>98%)and ethanol (dried,Wako Pure Chemical Co.,99.5%)were used for the synthesis of alkoxychlorosilanes.Tetramethylammonium hydroxide pen-tahydrate (Sigma-Aldrich,97%)and TEOS (Kishida Chemical Co.,>99%)were used to prepare D4R silicates.Poly(oxyeth-ylene)-poly(oxypropylene)-poly(oxyethylene)-type triblock co-polymer (EO 20PO 70EO 20,P123)was obtained from Sigma-Aldrich.Other chemicals,including pyridine (dried,Wako Pure Chemical Co.,99.5%),tetrahydrofuran (THF,dried,Wako Pure Chemical Industries,99.5%),hexane (Kanto Chemical Co.,96%),chloroform (Wako Pure Chemical Industries,99%),and 1N and 5N HCl (Wako Pure Chemical Industries)were used as received.Syntheses of Silylating Agents.Dimethylethoxychlorosi-lane [Me 2(EtO)SiCl],methyldiethoxychlorosilane [Me(EtO)2SiCl],and triethoxychlorosilane [(EtO)3SiCl]were synthesized by dropwise addition of ethanol to the corresponding chlorosilanes (molar ratios of ethanol/chlorosilanes were 1,2,and 3,respectively).The gaseous HCl generated by the reaction was allowed to go out of the vessel and was neutralized.The resulting liquids were the mixtures of silanes with various numbers of substituted alkoxyl groups,although monochloro-alkoxysilanes were dominant.After the removal of the species containing more than two Si -Cl groups under reduced pres-sure,the silylating agents [Me 2(EtO)SiCl,Me(EtO)2SiCl,and (EtO)3SiCl]containing 10,30,and 50%of completely alkoxy-lated species (Me 2Si(OEt)2,MeSi(OEt)3,and Si(OEt)4),respec-tively,were obtained as clear,colorless liquids.29Si NMR (99.3MHz,CDCl 3):δ13.3(Me 2(EtO)SiCl),-28.2(Me(EtO)2SiCl),and -70.3((EtO)3SiCl).Syntheses of Oligomeric Alkoxysilanes (1,2,and 3).D4R silicates (Si 8O 208-)were prepared from the mixture of TEOS/TMAOH/water/ethanol with the molar ratio of 1/1/10/10by vigorous stirring at room temperature for 3days.Hydrated crystals [(Me 4N)8Si 8O 20‚65H 2O]15were easily ob-tained by slow cooling of the concentrated D4R silicate solution.These crystals were dehydrated at 60°C under reduced pressure,yielding white powders.For alkoxysilylation,1g of this powder dissolved in 1.5mL of ethanol was added to the mixture of silylating agent,THF (100mL),and pyridine under vigorous stirring in an ice bath.The D4R/silylating agent/pyridine molar ratio was 1/150/10/0.Pyridine was added to neutralize HCl generated upon silylation.After the mixture was stirred for 30min,the unreacted silylating agents and solvents were removed under reduced pressure.The precipi-tates of pyridine hydrochloride were then removed by filtration to give a clear solution.Further removal of volatile components (mostly monomeric and dimeric alkoxysilanes)at 100°C under reduced pressure gave viscous liquids,from which the oligo-mers 1,2,and 3were finally isolated by gel permeation chromatography (GPC)using chloroform as the eluent.(9)(a)Fyfe,C.A.;Fu,G.J.Am.Chem.Soc.1995,117,9709.(b)Firouzi,A.;Kumar,D.;Bull,L.M.;Besier,T.;Sieger,P.;Huo,Q.;Walker,S.A.;Zasadzinski,J.A.;Glinka,C.;Nicol,J.;Margolese,D.;Stucky,G.D.;Chmelka,B.F.Science 1995,267,1138.(10)Zhang,L.;Abbenhuis,H. C.L.;Yang,Q.;Wang,Y.-M.;Magusin,P.C.M.M.;Mezari,B.;Santen,R.A.;Li,C.Angew.Chem.,Int.Ed.2007,46,5003.(11)(a)Hoebbel,D.;Reinert,T.;Endres,K.;Schmidt,H.Mater.Res.Soc.Symp.1994,346,863.(b)Hasegawa,I.;Ino,K.;Ohnishi,anomet.Chem.2003,17,287.(c)Hasegawa,I.;Motojima,anomet.Chem.1992,441,373.(12)Hasegawa,I.J.Sol -Gel Sci.Technol.1995,5,93.(13)Mochizuki,D.;Shimojima,A.;Kuroda,K.J.Am.Chem.Soc.2002,124,12082.(14)The synthesis procedures of dimeric and cubic octameric alkoxysilanes are contained in the Supporting Information.(15)Wiebcke,M.;Grube,M.;Koller,H.;Engelhardt,G.;Felsche,J.Microporous Mater.1993,2,55.Scheme 1.Novel Alkoxysilylated D4R Silicates (1,2and 3)as Nanobuilding Blocks of Xerogels (1G,2G,and 3G)and Mesostructured Films (1F,2F,and3F)B Chem.Mater.Hagiwara et al.Synthesis of Xerogels(1G,2G,and3G).Hydrolysis and polycondensation of the oligomers1,2,and3were conducted in the mixture of ethanol,H2O,and HCl.The mixtures were stirred at room temperature in closed vessels until gelation occurred.The molar ratio of oligomer/ethanol/H2O/HCl was 1/16/16/0.53.The xerogels1G,2G,and3G obtained by drying under a vacuum were pulverized before characterization.For comparison,xerogels were prepared by hydrolysis and poly-condensation of the1/1mixture of dimethyldiethoxysilane (DMDES)and TEOS,1/1mixture of methyltriethoxysialne (MTES)and TEOS,as well as TEOS alone.Synthesis of Mesostructured Films(1F,2F,and3F). The oligomers were partially hydrolyzed and polycondensed by stirring in the mixture of ethanol,H2O,and HCl at room temperature for1h.The mixtures were then added to the ethanol solution of a triblock copolymer surfactant(P123).The final molar ratio of the mixtures was1(or2or3)/ethanol/H2O/ HCl/P123)1/608/132/0.64/0.32.After being stirred for5min, the mixtures were spin-coated on glass substrates.Thin films were air-dried at room temperature for1day to afford as-synthesized films.The removal of the surfactant was carried out either by calcination at320°C for4h in air or by solventextraction(immersing the films in a stirred solution of ethanol (200mL)at room temperature for1day).Characterization.Liquid-state29Si NMR spectra were obtained on a JEOL Lambda-500spectrometer with resonance frequencies of99.25MHz.Solid-state29Si magic-angle spinning (MAS)NMR measurements were performed on a JEOL JNM-CMX-400spectrometer at a resonance frequency of79.42MHz, with a pulse width of45°and a recycle delay of100s. Deconvolution of the spectrum was performed by using a Gaussian function on Spinsight software,version4.3.2.Chemi-cal shifts for13C and29Si NMR were referenced to tetrameth-ylsilane at0ppm.Powder X-ray diffraction(XRD)patterns were recorded on a Mac Science M03XHF22diffractometer with Mn-filtered Fe K R radiation or on a Mac Science MXP3 diffractometer with Ni-filtered Cu K R radiation.Transmission electron microscopic(TEM)images of the mesostructured films were obtained on a JEOL JEM-2010microscope operated at 200kV.To prepare TEM samples,the films were scraped from substrates and ground with mortar and pestle and dispersed in ethanol.A carbon-coated copper grid was dipped in this dispersion and allowed to dry in air.FT-IR spectra of the products in KBr pellets were obtained using a Perkin-Elmer Spectrum One spectrometer with a nominal resolution of0.5 cm-1.Nitrogen adsorption measurements were performed by an Autosorb-1instrument(Quantachrome Instruments,Inc) at77K.Samples were preheated at120°C for3h under1×10-2Torr.Results and Discussion Alkoxysilylation of D4R silicate(Si8O208-).Hy-drated crystals of D4R silicate[(Me4N)8Si8O20‚65H2O]15 was dried at60°C under a vacuum prior to alkoxysi-lylation,which was crucial to minimize possible side reactions caused by water.Elemental analysis combined with thermogravimetry(TG)revealed that the H2O/D4R ratio decreased to about29.The solid-state29Si MAS NMR spectrum of these powders(see the Supporting Information,Figure S1)displayed a single signal at -96.3ppm(Q3unit),confirming that neither cleavage nor polycondensation of D4R units occurred during the drying process.Figure1shows the29Si NMR spectra of the oligomers 1,2,and3obtained by silylation of D4R silicate with alkoxychlorosilanes.The spectrum of1shows two signals at-9.9and-109.8ppm with the intensity ratio of1/1.These signals correspond to the D1and Q4units, respectively.16The oligomers2and3exhibit the T1 (-50.1ppm)and Q1(-89.4ppm)signals,16respectively,in addition to the Q4signals at around-110ppm.The presence of the Q4units strongly suggests that the (SiO)3SiOH(or O-)units of the D4R silicate(observed at-98ppm)were completely silylated without deterio-ration of the D4R units.The retention of the ethoxy groups without hydrolysis during silylation was con-firmed by13C NMR spectra(data not shown)that showed the signals of Si-O C H2C H3(59and18ppm). Thus the successful syntheses of1,2,and3are evident from these NMR data.Hydrolysis and Polycondensation Behavior.Hy-drolysis and polycondensation of1,2,and3led to gelation within several minutes,yielding transparent xerogels(1G,2G,and3G,respectively).The gelation time was much shorter than those of monomeric(Si-(OEt)4,TEOS)and dimeric((EtO)3Si-O-Si(OEt)3)alkox-ysilanes.14When these alkoxysilanes were reacted under the conditions at which the initial EtOH/Si,HCl/ Si,and H2O/SiOEt molar ratios were identical to those for synthesizing3G,gelation finally occurred after6and 3.5days of the reactions in the cases of monomeric and dimeric alkoxysilanes,respectively,even though13C NMR analysis revealed that ethoxy groups were almost completely hydrolyzed within1h(data not shown). Apparently,the larger molecular size of3allowed efficient growth of siloxane networks,resulting in extremely rapid gelation.The difference in the final EtOH/Si ratios in the systems after complete hydrolysis of ethoxy groups may also affect the gelation time. Actually,the ratio in the system of3(EtOH/Si)2.5) was relatively lower than those of monomer and dimer (EtOH/Si)5and4,respectively),thus favoring gela-tion.It should be noted,however,that gelation of3 occurred much faster(within6h)even when the molar ratio of ethanol was increased to the final EtOH/Si ratio of6.5.In addition to the molecular size,the siloxane struc-ture of the precursors had large effects on hydrolysis and polycondensation behavior.The reactivity of3 appeared to be much higher than the cubic octameric alkoxysilane(i.e.,ethoxy-derivative of D4R units).The(16)It is well-established that the29Si chemical shifts are largely influenced by the number of Si-C bonds and siloxane(Si-O-Si)bonds. ThesymbolsD n,T n,andQ n representC2Si(OSi)n(OX)2-n,C Si(OSi)n(OX)3-n, and Si(OSi)n(OX)4-n,where X)Et or H,respectively.Figure1.29Si NMR spectra of the oligomers:(a)1,(b)2, and(c)3in CDCl3.Building-Block Approach to Siloxane-Based Nanomaterials Chem.Mater.C13CNMR analysis of the hydrolysis process of cubic octameric alkoxysilane revealed that only 40%of alkoxy groups were hydrolyzed even after 1day of reaction.In this system,the amount of ethanol was increased to a final EtOH/Si ratio of 4,identical to that for the TEOS system,because of the lower solubility of octameric alkoxysilane (crystalline solids).Gelation did not occur even after 5days of reaction,which is in clear contrast to 3,the reaction of which proceeded so fast until gelation that we could not monitor by liquid-state NMR.Such a difference can be explained in terms of the hydrolysis mechanism;1,8hydrolysis of the cubic octamer should occur without inversion of the SiO 4tetrahedra,different from the S N 2type reaction potentially avail-able for 3.Thus we have demonstrated the specific feature of the oligomeric alkoxysilanes used in the present study,which is due to the large molecular size as well as the high reactivity of corner -Si(OEt)3groups.We expect that higher reaction rate of such oligomeric alkoxysi-lanes should contribute to minimization of the possible hydrolysis of Si -O -Si linkage during the reaction,which was previously reported for the methoxy-deriva-tive of D4R units.8Also important is that the use of oligomeric alkoxysi-lanes (1and 2),consisting of both SiO 4and methyl-substituted species (CSiO 3or C 2SiO 2),promises superior homogeneity of final xerogels if compared to that achieved by co-condensation of the mixture of mono-meric alkoxysilanes.Actually,the xerogel derived from the DMDES -TEOS mixture was visibly opaque,which suggests that phase separation occurred during the synthesis.In general,the reaction rate of alkoxysilanes is dominated by both inductive and steric effects.1Under acidic conditions,methyl-substituted alkoxysilanes such as DMDES and MTES are hydrolyzed faster than TEOS.It is therefore plausible to consider that each component is not homogeneously distributed in the final xerogel networks in the cases of the DMDES -TEOS and MTES -TEOS systems.Structures of Xerogels Prepared from 1,2,and 3.The formation of siloxane networks was confirmed by solid-state 29Si MAS NMR spectra of 1G ,2G ,and 3G (Figure 2).Note that structural modeling suggested that only intermolecular condensation was possible.Intramoleculer condensation between two alkoxysilyl groups to form a tetrasiloxane ring was strongly inhib-ited because of the large angle between the two Si -O bonds at the adjacent corners of a D4R unit.The spectrum of 1G (Figure 2a)mainly shows three signals corresponding to the D 1,D 2,and Q 4units.The D 2signal arises from the hydrolysis and condensation of -SiMe 2-(OEt)groups of 1to form siloxane linkages.From the D 2/(D 1+D 2)intensity ratio,it is estimated that 96%of the silyl groups at the cage corners are linked together.The spectrum of 2G consists of the signals correspond-ing to the T 1,T 2,and T 3units,along with the Q 4signal (Figure 2b).The (T 2+T 3)/(T 1+T 2+T 3)ratio indicates that 62%of the silyl groups of 2formed siloxane bonds.In the case of 3G ,broad Q 2,Q 3,and Q 4signals were observed (Figure 2c).The absence of the Q 1signal indicates that all of the terminal -Si(OEt)3groups of 3were hydrolyzed and polycondensed to form at least one siloxane bond.However,more detailed analysis of this spectrum is difficult because of the overlapping of the signals originating from the D4R units and the terminal -Si(OEt)3groups of 3.The Q 4signals observed for 1G and 2G are much narrower than those for the xerogels derived from the DMDES -TEOS and MTES -TEOS systems constituted of random siloxane networks (see the Supporting In-formation,Figure S2).This fact strongly suggests that the D4R units are retained in the networks of 1G and 2G .Although the small Q 3signals are indicative of the cleavage of Si -O -Si linkages during the reaction,their intensity ratios are smaller than those observed in Figure S2.In particular,the high Q 4/Q 3ratio of 2G is in clear contrast to the MTES -TEOS system where the large Q 3signals and even Q 2signal are observed.Also interesting is that the T 3/T 2ratios for 2G is much smaller than for the xerogels derived from the MTES -TEOS system.This indicates that the formation of three Si -O -Si linkage is sterically restricted.But unfortu-nately,we cannot see any difference in the spectra of 3G and TEOS-derived xerogel.Further information on the siloxane networks was obtained by FT-IR.Figure 3shows the FT-IR spectra of 1G ,2G ,and 3G .All of the samples show the absorption bands due to siloxane frameworks at around 400-450,800,and 1000-1200cm -1,1consistentwithFigure 2.Solid-state 29Si MAS NMR spectra of the xerogels:(a)1G ,(b)2G ,and (c)3G.Figure 3.FT-IR spectra of the xerogels:(a)1G ,(b)2G ,and (c)3G .D Chem.Mater.Hagiwara et al.the 29Si MAS NMR data.Also,the band due to Si -OH is observed at around 950cm -1.This peak becomes more prominent as the number of alkoxy groups of the oligomers increases (i.e.,1G <2G <3G ).This is in agreement with the 29Si NMR data showing that 3G has a certain amount of the Q 3units,whereas alkoxy groups in 1G were fully hydrolyzed and polycondensed to form the D 2units.The minor differences in the shapes and positions of these bands can be attributed to the differences in the arrangement of siloxane networks and to the number of methyl groups.It is important to note here that 1G ,2G ,and 3G exhibit characteristic bands at 550cm -1that are apparently larger than for the xerogels prepared from the mixture of the monomeric alkoxysilanes (see the Supporting Information,Figure S3).This band is reported to be associated with the vibration of D4R units.10,17This fact,together with the 29Si MAS NMR data showing the presence of the Q 4units as the dominant species,strongly suggests that D4R units are retained in the xerogel networks.Figure 4shows the powder XRD patterns of the xerogels 1G ,2G ,and 3G .All of the samples exhibit broad diffraction peaks at around 2θ)25+(d )0.3nm),which is typically observed for amorphous silica.Additional peaks also appear at lower scattering angle (d ≈1.1nm)in the patterns of 2G and 3G ,suggesting a certain structural periodicity in these products.Such diffraction peaks have previously been observed for the XRD patterns of xerogels prepared by the reaction of D4R silicates with dimethydichlorosilanes.12However,these peaks are also observable for the xerogels derived from the DMDES -TEOS and MTES -TEOS mixtures (see the Supporting Information,Figure S4).We there-fore suppose that the structural periodicities are not associated with the D4R units,but they may be at-tributed to some ring structures,such as tetrasiloxane rings and ladder polymers,although the details are still unclear.Porosity of Xerogels (1G,2G,and 3G).The num-ber of alkoxy groups in the oligomers is critical to the porosity of the resulting silica-based xerogels.Figure 5shows the nitrogen adsorption isotherms of 1G ,2G ,and 3G .The isotherm of 2G displays a type I curve,typicalof microporous silica.The Brunauer -Emmett -Teller (BET)surface area and Saito -Foley (SF)pore diameter were calculated to be 600m 2g -1and ca.1nm,respectively.In contrast,1G and 3G show no increase of the nitrogen uptake,suggesting that they are non-porous or have only closed pores.Such a difference should reflect the variation in the siloxane networks,and two Si -OEt groups at one cage corner are ap-propriate to generate pores in the products.In the case of 1G ,the oligomer 1is capable of forming only one siloxane bond at each corner of the D4R unit,resulting in the formation of relatively “flexible”net-works that possibly undergo large shrinkage during drying process.Previously,Harrison and co-worker reported the xerogel with high surface area (218.3m 2g -1)prepared from monobromosilylated-D4R units,which is similar to 1with respect to the number of reactive sites.7b Such a difference is possibly due to the difference in the reactivities of Si -Br and Si -OEt groups.On the other hand,the oligomer 3possesses 24functional groups (Si -OEt)and is therefore expected to form rather “dense”networks,leaving no void spaces between the D4R units accessible for N 2molecules.18This is an unusual phenomenon in sol -gel chemistry because acid-catalyzed hydrolysis and polycondensation of tetraalkoxysilanes generally yield porous silica xe-rogels.Actually,monomeric and dimeric alkoxysilanes afforded porous silicas with the BET surface area of 560m 2g -1and 490m 2g -1,respectively.Also,it is reported that cubic octameric alkoxysilane gave microporous silica by hydrolysis and polycondensation.8Thus these results open a way for the rational control of the porosity of silica-based materials by molecular design.Surfactant-Directed Synthesis of Mesostruc-tured Films (1F,2F,and 3F).The oligomeric alkox-ysilanes 2and 3can be used for producing hierarchical structures.Thin films (1F ,2F ,and 3F )obtained by hydrolysis and polycondensation of 1,2,and 3in the presence of a surfactant (P123)were transparent with the thickness of ca.200nm.Figure 6shows the XRD(17)Huang,Y.;Jiang,Z.Microporous Mater.1997,12,341.(18)It should be noted that the porosity of the xerogel derived from 3is not only dominated by the molecular structure of 3but also appears to be affected by reaction conditions (such as the amount of solvent and the drying conditions).Detailed study isunderway.Figure 4.Powder XRD patterns of the xerogels:(a)1G ,(b)2G ,and (c)3G.Figure 5.Nitrogen adsorption -desorption isotherms of (a)1G ,(b)2G ,and (c)3G .The open symbols and the filled symbols denote adsorption and desorption,respectively.Building-Block Approach to Siloxane-Based Nanomaterials Chem.Mater.Epatterns of the films.Although 1F has no ordered structure,2F and 3F exhibit sharp diffraction peaks with the d spacings of 9.3and 8.8nm,respectively,accompanying second-order reflections.Because these periodic structures were still retained even after calci-nation (d )5.1and 4.7nm,Figure 7),these films should possess three-dimensional siloxane frameworks rather than a lamellar structure that usually collapses upon surfactant removal.The TEM images of 2F after calcination mainly shows striped patterns suggestive of a 2D hexagonal structure (Figure 8a).The two XRD peaks are therefore indexed as 10and 20reflections.The lack of a (11)peak suggests that the mesochannels are oriented parallel to the substrate surface.19How-ever,the presence of the domain with a wormholelike structure was also confirmed (Figure 8b).The formation of mesostructured silica by using amphiphilic block copolymers having poly(ethylene oxide)(PEO)chains as hydrophilic blocks relies on the noncovalent interactions between silanol groups and PEO blocks.20,21The lack of structural order in 1F is possibly due to an insufficient interaction of hydrolyzed 1with PEO blocks of the surfactant,because of thesmaller number of Si -OH groups (only 0.5SiOH per SiO 4).Also,the relatively hydrophobic nature of 1having 16terminal methyl groups should be unfavor-able for the partitioning of the oligomeric units into the hydrophilic PEO regions of liquid-crystalline phases during the evaporation-induced self-assembly process.22To minimize the possible thermal rearrangement of the siloxane networks,we typically performed solvent extraction of the surfactants for the film 2F .Figure 9compares the IR spectra of as-synthesized 2F with those of 2F after calcination and after solvent extraction.The absorption bands due to the C -H stretching modes (2800-3000cm -1)considerably decreased by either calcination or solvent extraction.The molar ratio of Si -CH 3groups and -CH 3groups of the PPO blocks is roughly estimated to be 2/5from the 2/P123ratio in the starting solution.We suppose that the remaining bands observed after calcination or solvent extraction are mostly ascribed to the Si -CH 3groups.Upon surfactant removal,the d spacings of the (10)peaks significantly decreased because of the shrinkage of the siloxane frameworks (Figure 7).Nevertheless,the IR spectrum of the film after solvent-extraction clearly shows the band at 550cm -1,suggesting the retention of the D4R units,in contrast to the calcined film exhibiting a relatively ill-defined band at this region.Thus we clearly demonstrated the successful synthesis of hybrid meso-porous silica films containing D4R units in the frame-works.(19)Miyata,H.;Kuroda,K.Chem.Mater.1999,11,1609.(20)Soler-Illia,G.J.d.A.A.;Crepaldi,E.L.;Grosso,D.;Sanchez,C.Curr.Opin.Colloid Interface Sci.2003,8,109.(21)Soler-Illia,G.J.d.A.A.;Innocenzi,P.Chem.s Eur.J 2006,12,4478.(22)Brinker,C.J.;Lu,Y.;Sellinger,A.;Fan,H.Adv.Mater.1999,11,579.Figure 6.XRD patterns of the films:(a)1F ,(b)2F ,and (c)3F.Figure 7.XRD patterns of (a)2F after calcination and (b)2F aftersolvent-extraction.Figure 8.TEM images of 2F aftercalcination.Figure 9.FT-IR spectra of:(a)as-synthesized 2F ,(b)2F after calcination,and (c)2F after solvent-extraction.F Chem.Mater.Hagiwara et al.。