Remediation of soil contaminated with lubricating oil by extraction
重金属污染土壤修复后资源化利用制备陶粒

随着经济的快速发展,工业化和城市化的进程加快,许多工业企业通过关闭、破产、异地迁建等陆续搬出主城区,原有的工业企业用地被逐调整为居住用地或公建用地。
由于历史上的粗放型生产导致场地土壤被不同程度的污染,需要通过对企业拆迁污染场地风险评估和修复治理来实现净地转移。
随着2016年以来,国务院颁布《土壤污染治理防治行动计划》的实施,各省也陆续出台了针对污染土壤治理修复的相关政策文件,近年来,各省(区、市)相继开展了一系列的污染场地治理修复工程,据土壤修复行业市场规模数据显示,2017年~2021年我国土壤修复行业公开招标项目数量分别为800个、1468个、1698个、3521个、3626个,土壤环境修复项目数量逐年增加,其中尤以2020年增加幅度最为明显,2021年持续增长。
在政策导向、场地再开发利用需求和环保意识增强的影响下,土壤修复工程行业受到持续关注。
以2021年为例,全国钢铁行业遗留场地修复工程项目共启动15个,主要在重庆、杭州、安徽等地,项目合同额总计达30亿元,占全国修复工程金额的25%。
其中围绕着重钢、杭钢和合肥马钢等三个大型污染地块共计产生了25.2亿元的修复工程,占全国总修复工程市场的五分之一。
因此,重金属污染土壤修复治理及修复后土壤和土地资源的再利用,已成为国内城镇环境治理与开发的热点。
而以重金属污染土壤为原料,用于制备建材陶粒,不仅能有效地减小重金属污染土对环境的危害,还能起到变废为宝,提高社会效益及经济效益的作用。
1重金属污染土壤1.1概念重金属一般是指密度在5.0g/cm 3以上的45种元素,在环境污染研究中,通常所说的土壤重金属污染所指的重金属,主要是指Zn 、Cu 、Cr 、Cd 、Pb 、Ni 、Hg 以及类金属As ,8种重金属引起的土壤污染。
土壤重金属污染是指由于人类活动将重金属直接或者间接地引入土壤中,导致土壤中重金属明显高于原生含量,并造成生态环境质量恶化的现象。
污染土壤生物修复技术的进展与工程应用现状

化工进展Chemical Industry and Engineering Progress2023 年第 42 卷第 12 期污染土壤生物修复技术的进展与工程应用现状房晓宇,卢滇楠,刘铮(清华大学化学工程系,北京 100084)摘要:人类生产和生活中对于污染物的不当处理会导致土壤污染,威胁生态安全、粮食安全和可持续发展。
土壤生物修复利用微生物来降解土壤中的有机污染物、转化重金属污染物价态或者降低其生物可利用度而降低其危害。
伴随现代生物技术的发展,土壤生物修复技术被日益广泛地应用于污染耕地和污染工业场地的修复。
本文从污染物质的转化与利用角度,概述了土壤污染物的主要类型及其所适用的生物修复技术及其进展。
重点综述了生物修复菌株的筛选、土壤微生态分析、生物修复过程强化三方面的最新进展,介绍了生物修复技术在加油站、废弃化工厂的生物修复及秸秆还田中的工程实施案例,分析了土壤生物修复技术应用中存在的问题,如土壤修复效果评估和降解菌剂性能强化等,讨论了土壤生物修复技术的研究方向和应用前景。
关键词:污染土壤;土壤生物修复;废弃秸秆中图分类号:TQ033 文献标志码:A 文章编号:1000-6613(2023)12-6498-09Recent advancements and applications of soil bioremediation techniquesFANG Xiaoyu ,LU Diannan ,LIU Zheng(Department of Chemical Engineering, Tsinghua University, Beijing 100084, China)Abstract: Soil contamination is often caused by the inappropriate treatment of industrial wastes andmunicipal sewage threatening the safety of environment, food and ecology as well as the sustainability of society. Bioremediation refers to the application of microorganisms to dissociate organic compounds, detoxifying heavy metal ions or reducing their bioavailability. The advancement of biotechnology has empowered technical innovation of bioremediation methods and their applications in the treatment ofcontaminated farmland and wasted plant site. This review starts with a brief introduction to bioremediationtechniques and their applications to three major types of soil contaminants. The applicability of these methods was discussed from the viewpoint of contaminates transformation and utilization. The technical advancement in the selection and screening of degradation microorganisms, molecular biology methods for assessing microbiological ecology as well as novel bioaugmentation principles were detailed. The applications of bioremediation techniques in the treatment of gas stations, abandoned plants and straw mulching were described. The problems in the development of soil bioremediation techniques such as the assessment of soil remediation outcome, formation of high performance degrading microbial consortia wereoutlined, as well as the prospects of soil remediation techniques.Keywords: contaminated soil; soil bioremediation; straw综述与专论DOI :10.16085/j.issn.1000-6613.2023-0046收稿日期:2023-01-10;修改稿日期:2023-02-20。
新托福TPO5阅读原文(一)Minerals and Plants及译文
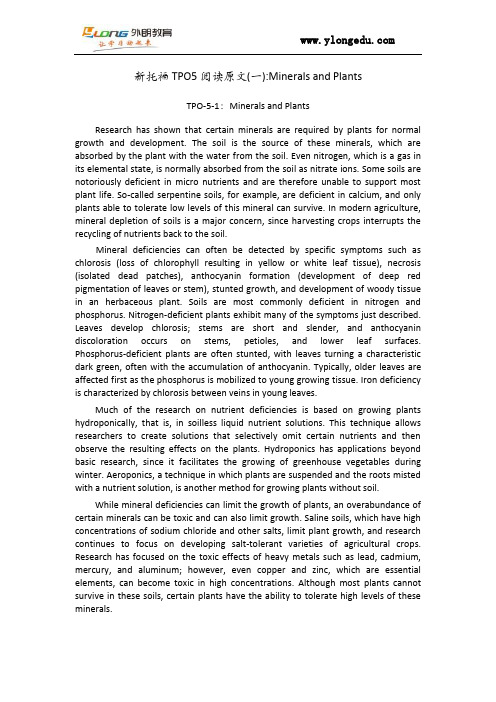
新托福TPO5阅读原文(一):Minerals and PlantsTPO-5-1:Minerals and PlantsResearch has shown that certain minerals are required by plants for normal growth and development. The soil is the source of these minerals, which are absorbed by the plant with the water from the soil. Even nitrogen, which is a gas in its elemental state, is normally absorbed from the soil as nitrate ions. Some soils are notoriously deficient in micro nutrients and are therefore unable to support most plant life. So-called serpentine soils, for example, are deficient in calcium, and only plants able to tolerate low levels of this mineral can survive. In modern agriculture, mineral depletion of soils is a major concern, since harvesting crops interrupts the recycling of nutrients back to the soil.Mineral deficiencies can often be detected by specific symptoms such as chlorosis (loss of chlorophyll resulting in yellow or white leaf tissue), necrosis (isolated dead patches), anthocyanin formation (development of deep red pigmentation of leaves or stem), stunted growth, and development of woody tissue in an herbaceous plant. Soils are most commonly deficient in nitrogen and phosphorus. Nitrogen-deficient plants exhibit many of the symptoms just described. Leaves develop chlorosis; stems are short and slender, and anthocyanin discoloration occurs on stems, petioles, and lower leaf surfaces. Phosphorus-deficient plants are often stunted, with leaves turning a characteristic dark green, often with the accumulation of anthocyanin. Typically, older leaves are affected first as the phosphorus is mobilized to young growing tissue. Iron deficiency is characterized by chlorosis between veins in young leaves.Much of the research on nutrient deficiencies is based on growing plants hydroponically, that is, in soilless liquid nutrient solutions. This technique allows researchers to create solutions that selectively omit certain nutrients and then observe the resulting effects on the plants. Hydroponics has applications beyond basic research, since it facilitates the growing of greenhouse vegetables during winter. Aeroponics, a technique in which plants are suspended and the roots misted with a nutrient solution, is another method for growing plants without soil.While mineral deficiencies can limit the growth of plants, an overabundance of certain minerals can be toxic and can also limit growth. Saline soils, which have high concentrations of sodium chloride and other salts, limit plant growth, and research continues to focus on developing salt-tolerant varieties of agricultural crops. Research has focused on the toxic effects of heavy metals such as lead, cadmium, mercury, and aluminum; however, even copper and zinc, which are essential elements, can become toxic in high concentrations. Although most plants cannot survive in these soils, certain plants have the ability to tolerate high levels of these minerals.Scientists have known for some time that certain plants, called hyperaccumulators, can concentrate minerals at levels a hundredfold or greater than normal. A survey of known hyperaccumulators identified that 75 percent of them amassed nickel, cobalt, copper, zinc, manganese, lead, and cadmium are other minerals of choice. Hyperaccumulators run the entire range of the plant world. They may be herbs, shrubs, or trees. Many members of the mustard family, spurge family, legume family, and grass family are top hyperaccumulators. Many are found in tropical and subtropical areas of the world, where accumulation of high concentrations of metals may afford some protection against plant-eating insects and microbial pathogens.Only recently have investigators considered using these plants to clean up soil and waste sites that have been contaminated by toxic levels of heavy metals–an environmentally friendly approach known as phytoremediation. This scenario begins with the planting of hyperaccumulating species in the target area, such as an abandoned mine or an irrigation pond contaminated by runoff. Toxic minerals would first be absorbed by roots but later relocated to the stem and leaves. A harvest of the shoots would remove the toxic compounds off site to be burned or composted to recover the metal for industrial uses. After several years of cultivation and harvest, the site would be restored at a cost much lower than the price of excavation and reburial, the standard practice for remediation of contaminated soils. For examples, in field trials, the plant alpine pennycress removed zinc and cadmium from soils near a zinc smelter, and Indian mustard, native to Pakistan and India, has been effective in reducing levels of selenium salts by 50 percent in contaminated soils.译文:TPO-5-1 矿物质和植物研究表明,某些矿物质是植物正常生长发育所必需的。
硫代硫酸盐浸提法修复汞污染土壤及含汞浸出液的光分解回收处理

'f e 知库Eco-Environmental Knowledge Web^v v w ) 环境工程学报Chinese Journal ofEnvironmental Engineering(^3^1 E-mail:*************.cn第14卷第12期2020年12月 Vol . 14, No .12 Dec . 2020齊(010) 62941074文章栏目:土壤污染防治DOI10.12030/j.cjee.201911030中图分类号X53文献标识码A畅永锋,梁鹏,路殿坤,等.硫代硫酸盐浸提法修复汞污染土壤及含汞浸出液的光分解回收处理[J].环境工程学报,2020,14(12): 3527-3533.C H A N G Y o n g f e n g , L I A N G P e n g , L UD i a n k u n , et al . Remediation of m e r cury contaminated soil with thiosulfate solution washing followed b y photo-decomposing and separating mercury fro m w a s hing solution [J ]. Chinese Journal of Environmental Engineering , 2020, 14(12): 3527-3533.硫代硫酸盐浸提法修复汞污染土壤及含汞浸出液的光分解回收处理畅永锋,梁鹏,路殿坤,谢锋*东北大学冶金学院,沈阳110819第一作者:畅永锋(1977—),男,博士,讲师。
研究方向:固体废弃物处理。
E -m a i l :changyf @s m m .n e u *通信作者:谢锋(1970—),男,博士,教授。
研究方向:清洁生产及固废资源化。
E -m a i l : xief @s m m .n e u 摘要汞污染土壤对环境的危害巨大,已成为国内外主要关注的环境问题之一。
砷污染土壤修复技术综述

砷污染土壤修复技术综述一、本文概述Overview of this article随着工业化、城市化进程的加快,土壤砷污染问题日益凸显,对生态环境和人类健康构成了严重威胁。
砷是一种有毒元素,长期摄入会对人体造成多系统、多脏器的损害。
因此,开展砷污染土壤修复技术的研究与应用,对于保护生态环境、维护人类健康具有重要意义。
With the acceleration of industrialization and urbanization, the problem of soil arsenic pollution is becoming increasingly prominent, posing a serious threat to the ecological environment and human health. Arsenic is a toxic element, and long-term intake can cause damage to multiple systems and organs in the human body. Therefore, conducting research and application of arsenic contaminated soil remediation technology is of great significance for protecting the ecological environment and maintaining human health.本文旨在对砷污染土壤修复技术进行全面综述,总结当前国内外在该领域的研究进展和技术应用情况。
文章首先介绍了砷污染土壤的来源、分布及危害,然后重点阐述了各种砷污染土壤修复技术的原理、优缺点及适用范围,包括物理修复、化学修复、生物修复等方法。
文章对未来砷污染土壤修复技术的发展趋势和研究方向进行了展望,以期为相关领域的研究和实践提供有益的参考和借鉴。
加油站场地调查及污染土壤和地下水修复方法研究
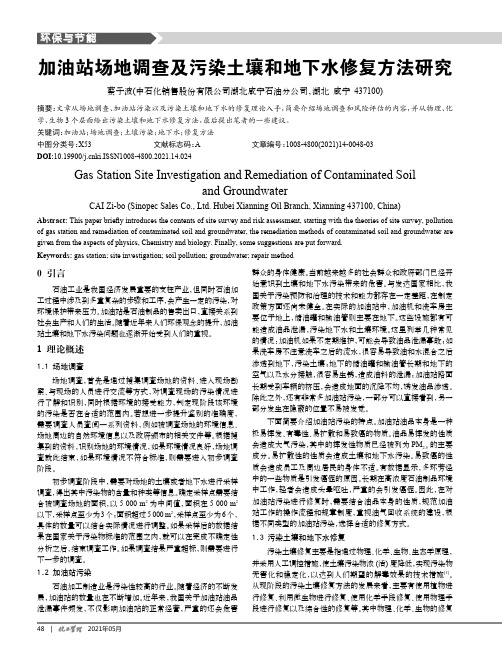
群众的身体健康。
当前越来越多的社会群众和政府部门已经开始意识到土壤和地下水污染带来的危害。
与发达国家相比,我国关于污染预防和治理的技术和能力都存在一定差距,在制定政策方面还尚未健全。
在实际的加油站中,加油机和洗车房主要位于地上,储油罐和输油管则主要在地下。
这些设施都有可能造成油品泄漏,污染地下水和土壤环境。
这里列举几种常见的情况:加油机如果不定期维护,可能会导致油品泄漏事故;如果洗车房不注意洗车之后的流水,很容易导致油和水混合之后渗透到地下,污染土壤;地下的储油罐和输油管长期和地下的空气以及水分接触,很容易生锈,造成油料的泄漏;加油站路面长期受到车辆的挤压,会造成地面的沉降不均,诱发油品渗透。
除此之外,还有非常多加油站污染,一部分可以直接看到,另一部分发生在隐蔽的位置不易被发觉。
下面简要介绍加油站污染的特点。
加油站油品本身是一种极易挥发、有毒性、易扩散和易致癌的物质。
油品易挥发的性质会造成大气污染,其中的挥发性物质已经被列为PM 2.5的主要成分。
易扩散性的性质会造成土壤和地下水污染。
易致癌的性质会造成员工及周边居民的身体不适。
有数据显示,多环芳烃中的一些物质是引发癌症的原因。
长期在高浓度石油制品环境中工作,轻者会造成头晕呕吐,严重的会引发癌症。
因此,在对加油站污染进行修复时,需要结合油品本身的性质,规范加油站工作的操作流程和规章制度,重视油气回收系统的建设,根据不同类型的加油站污染,选择合适的修复方式。
1.3 污染土壤和地下水修复污染土壤修复主要是指通过物理、化学、生物、生态学原理,并采用人工调控措施,使土壤污染物浓(活)度降低,实现污染物无害化和稳定化,以达到人们期望的解毒效果的技术措施[1]。
从现阶段的污染土壤修复方法的发展来看,主要有使用植物进行修复、利用微生物进行修复、使用化学手段修复、使用物理手段进行修复以及综合性的修复等。
其中物理、化学、生物的修复0 引言石油工业是我国经济发展重要的支柱产业,但同时石油加工过程中涉及到多重复杂的步骤和工序,会产生一定的污染,对环境保护带来压力。
正向推理法快速解决托福阅读推断题

正向推理法快速解决托福阅读推断题推理题作犯难度较高简单出错的题型,我整理了一些应对推理题的小攻略,下面我就和大家共享,来观赏一下吧。
托福阅读攻略:推理题推理“度”的把握TPO的做题阅历告知我们,推理题的答案既要防止“推理过度”,又要当心“推理不足”,这个度拿捏不好,推理题的正确率就不高。
题目分类我们可以把它的题目分为两类。
一类是“题干定位型”,一类是“选项定位型”。
题干定位题,顾名思义,就是通过题目中的词可以定位到原文中答案所在的位置从而选出答案;而选项定位型则是指无法通过题目来定位的状况,比如说:题目问What can be inferred about stingless bees ?而原文中整段都在讲stingless bees,就没有方法去详细定位,这个时候就可以利用选项去定位原文。
反向推理今日我们来介绍一种解题方法——反向推理。
在学术写作中,当我们将事物根据某种标准进行分类后,分类的内容既不重合也不丢失。
比如我们把A分成X和Y,X和Y之间没有重合的部分,同时X和Y合并构成A,也不会丢失A的某一部分。
这样一来,当我们知道X所具有某一个特性,就能推出Y不具有这样的特性,这就是反向推理。
TPO例题A symbiotic relationship is an interaction between two or more species in which one species lives in or on another species. There are three main types of symbiotic relationships: parasitism, commensalism, and mutualism. The first and the third can be key factors in the structure of a biological community; that is, all the populations of organisms living together and potentially interacting in a particular area.Which of the following statements about commensalism can be inferred from paragraph 1?A.It excludes interactions between more than two species.B.It makes it less likely for species within a community, to survive.C.Its significance to the organization of biological communities is small.D.Its role in the structure of biological populations is a disruptive one.题目解析依据题干的commensalism, 可以找到定位区间There are three main types of symbiotic relationships: parasitism, commensalism, and mutualism. The first and the third can be key factors in the structure of a biological community。
土壤退化与修复技术的研究进展参考文献
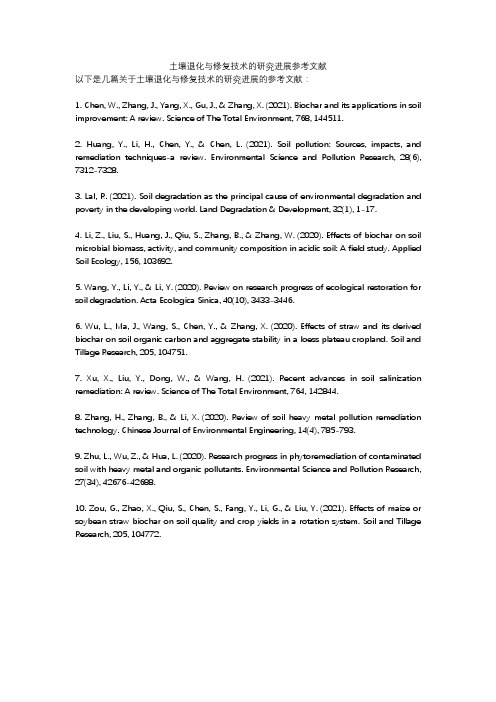
土壤退化与修复技术的研究进展参考文献以下是几篇关于土壤退化与修复技术的研究进展的参考文献:1. Chen, W., Zhang, J., Yang, X., Gu, J., & Zhang, X. (2021). Biochar and its applications in soil improvement: A review. Science of The Total Environment, 768, 144511.2. Huang, Y., Li, H., Chen, Y., & Chen, L. (2021). Soil pollution: Sources, impacts, and remediation techniques-a review. Environmental Science and Pollution Research, 28(6), 7312-7328.3. Lal, R. (2021). Soil degradation as the principal cause of environmental degradation and poverty in the developing world. Land Degradation & Development, 32(1), 1-17.4. Li, Z., Liu, S., Huang, J., Qiu, S., Zhang, B., & Zhang, W. (2020). Effects of biochar on soil microbial biomass, activity, and community composition in acidic soil: A field study. Applied Soil Ecology, 156, 103692.5. Wang, Y., Li, Y., & Li, Y. (2020). Review on research progress of ecological restoration for soil degradation. Acta Ecologica Sinica, 40(10), 3433-3446.6. Wu, L., Ma, J., Wang, S., Chen, Y., & Zhang, X. (2020). Effects of straw and its derived biochar on soil organic carbon and aggregate stability in a loess plateau cropland. Soil and Tillage Research, 205, 104751.7. Xu, X., Liu, Y., Dong, W., & Wang, H. (2021). Recent advances in soil salinization remediation: A review. Science of The Total Environment, 764, 142844.8. Zhang, H., Zhang, B., & Li, X. (2020). Review of soil heavy metal pollution remediation technology. Chinese Journal of Environmental Engineering, 14(4), 785-793.9. Zhu, L., Wu, Z., & Hua, L. (2020). Research progress in phytoremediation of contaminated soil with heavy metal and organic pollutants. Environmental Science and Pollution Research, 27(34), 42676-42688.10. Zou, G., Zhao, X., Qiu, S., Chen, S., Fang, Y., Li, G., & Liu, Y. (2021). Effects of maize or soybean straw biochar on soil quality and crop yields in a rotation system. Soil and Tillage Research, 205, 104772.。
英文文献小短文(原文加汉语翻译)
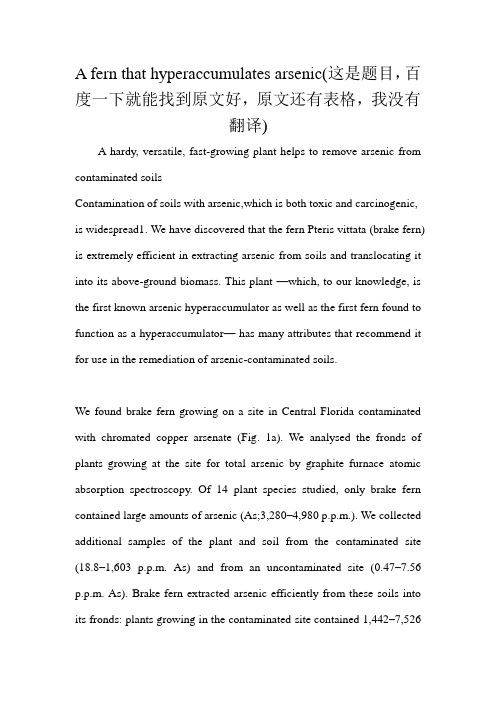
A fern that hyperaccumulates arsenic(这是题目,百度一下就能找到原文好,原文还有表格,我没有翻译)A hardy, versatile, fast-growing plant helps to remove arsenic from contaminated soilsContamination of soils with arsenic,which is both toxic and carcinogenic, is widespread1. We have discovered that the fern Pteris vittata (brake fern) is extremely efficient in extracting arsenic from soils and translocating it into its above-ground biomass. This plant —which, to our knowledge, is the first known arsenic hyperaccumulator as well as the first fern found to function as a hyperaccumulator— has many attributes that recommend it for use in the remediation of arsenic-contaminated soils.We found brake fern growing on a site in Central Florida contaminated with chromated copper arsenate (Fig. 1a). We analysed the fronds of plants growing at the site for total arsenic by graphite furnace atomic absorption spectroscopy. Of 14 plant species studied, only brake fern contained large amounts of arsenic (As;3,280–4,980 p.p.m.). We collected additional samples of the plant and soil from the contaminated site (18.8–1,603 p.p.m. As) and from an uncontaminated site (0.47–7.56 p.p.m. As). Brake fern extracted arsenic efficiently from these soils into its fronds: plants growing in the contaminated site contained 1,442–7,526p.p.m. Arsenic and those from the uncontaminated site contained 11.8–64.0 p.p.m. These values are much higher than those typical for plants growing in normal soil, which contain less than 3.6 p.p.m. of arsenic3.As well as being tolerant of soils containing as much as 1,500 p.p.m. arsenic, brake fern can take up large amounts of arsenic into its fronds in a short time (Table 1). Arsenic concentration in fern fronds growing in soil spiked with 1,500 p.p.m. Arsenic increased from 29.4 to 15,861 p.p.m. in two weeks. Furthermore, in the same period, ferns growing in soil containing just 6 p.p.m. arsenic accumulated 755 p.p.m. Of arsenic in their fronds, a 126-fold enrichment. Arsenic concentrations in brake fern roots were less than 303 p.p.m., whereas those in the fronds reached 7,234 p.p.m.Addition of 100 p.p.m. Arsenic significantly stimulated fern growth, resulting in a 40% increase in biomass compared with the control (data not shown).After 20 weeks of growth, the plant was extracted using a solution of 1:1 methanol:water to speciate arsenic with high-performance liquid chromatography–inductively coupled plasma mass spectrometry. Almostall arsenic was present as relatively toxic inorganic forms, with little detectable organoarsenic species4. The concentration of As(III) was greater in the fronds (47–80%) than in the roots (8.3%), indicating that As(V) was converted to As(III) during translocation from roots to fronds.As well as removing arsenic from soils containing different concentrations of arsenic (Table 1), brake fern also removed arsenic from soils containing different arsenic species (Fig. 1c). Again, up to 93% of the arsenic was concentrated in the fronds. Although both FeAsO4 and AlAsO4 are relatively insoluble in soils1, brake fern hyperaccumulated arsenic derived from these compounds into its fronds (136–315 p.p.m.)at levels 3–6 times greater than soil arsenic.Brake fern is mesophytic and is widely cultivated and naturalized in many areas with a mild climate. In the United States, it grows in the southeast and in southern California5. The fern is versatile and hardy, and prefers sunny (unusual for a fern) and alkaline environments (where arsenic is more available). It has considerable biomass, and is fast growing, easy to propagate,and perennial.We believe this is the first report of significant arsenic hyperaccumulationby an unmanipulated plant. Brake fern has great potential to remediate arsenic-contaminated soils cheaply and could also aid studies of arsenic uptake, translocation, speciation, distribution and detoxification in plants. *Soil and Water Science Department, University ofFlorida, Gainesville, Florida 32611-0290, USAe-mail: lqma@†Cooperative Extension Service, University ofGeorgia, Terrell County, PO Box 271, Dawson,Georgia 31742, USA‡Department of Chemistry & SoutheastEnvironmental Research Center, FloridaInternational University, Miami, Florida 33199,1. Nriagu, J. O. (ed.) Arsenic in the Environment Part 1: Cyclingand Characterization (Wiley, New York, 1994).2. Brooks, R. R. (ed.) Plants that Hyperaccumulate Heavy Metals (Cambridge Univ. Press, 1998).3. Kabata-Pendias, A. & Pendias, H. in Trace Elements in Soils and Plants 203–209 (CRC, Boca Raton, 1991).4. Koch, I., Wang, L., Ollson, C. A., Cullen, W. R. & Reimer, K. J. Envir. Sci. Technol. 34, 22–26 (2000).5. Jones, D. L. Encyclopaedia of Ferns (Lothian, Melbourne, 1987).积累砷的蕨类植物耐寒,多功能,生长快速的植物,有助于从污染土壤去除砷有毒和致癌的土壤砷污染是非常广泛的。
化学与社会课程论文
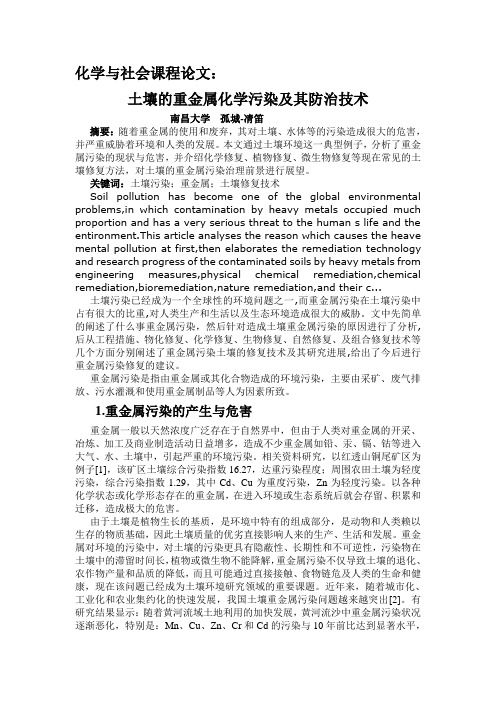
化学与社会课程论文:土壤的重金属化学污染及其防治技术南昌大学孤城-凊笛摘要:随着重金属的使用和废弃,其对土壤、水体等的污染造成很大的危害,并严重威胁着环境和人类的发展。
本文通过土壤环境这一典型例子,分析了重金属污染的现状与危害,并介绍化学修复、植物修复、微生物修复等现在常见的土壤修复方法,对土壤的重金属污染治理前景进行展望。
关键词:土壤污染;重金属;土壤修复技术Soil pollution has become one of the global environmental problems,in which contamination by heavy metals occupied much proportion and has a very serious threat to the human s life and the entironment.This article analyses the reason which causes the heave mental pollution at first,then elaborates the remediation technology and research progress of the contaminated soils by heavy metals from engineering measures,physical chemical remediation,chemical remediation,bioremediation,nature remediation,and their c...土壤污染已经成为一个全球性的环境问题之一,而重金属污染在土壤污染中占有很大的比重,对人类生产和生活以及生态环境造成很大的威胁。
文中先简单的阐述了什么事重金属污染,然后针对造成土壤重金属污染的原因进行了分析,后从工程措施、物化修复、化学修复、生物修复、自然修复、及组合修复技术等几个方面分别阐述了重金属污染土壤的修复技术及其研究进展,给出了今后进行重金属污染修复的建议。
钢渣施用对多金属复合污染土壤的改良效果及水稻吸收重金属的影响

农业环境科学学报2011,30(3):455-460Journal of Agro-Environment Science钢渣施用对多金属复合污染土壤的改良效果及水稻吸收重金属的影响邓腾灏博1,谷海红1,仇荣亮1,2*(1.中山大学环境科学与工程学院,广州510275;2.广东省环境污染控制与修复技术重点实验室,广州510275)摘要:通过盆栽实验和大田实验研究了钢渣施用对多金属复合污染土壤pH、有效硅、有效态重金属含量(镉、铅、铜、锌)以及水稻吸收重金属的影响。
盆栽实验设置5个处理,分别是CK(无钢渣)、SS3和SS6(分别加入3g·kg-1和6g·kg-1100目钢渣)、FSS3和FSS6(分别加入3g·kg-1和6g·kg-1180目钢渣);大田实验设置两个处理:CK(无钢渣)和SS(加入3g·kg-1100目钢渣)。
结果表明,盆栽实验土壤pH值及有效硅含量随钢渣施用量的增加和粒径的减小显著上升,土壤有效态重金属含量则出现显著下降。
水稻地上部重金属浓度在施加钢渣后均显著降低,并且远低于地下部浓度;大部分钢渣施加处理对降低地下部重金属浓度也有显著效果。
大田实验结果显示,钢渣改良的处理,不仅提高了水稻产量,稻米中的重金属浓度也得到了大幅降低,并达到了国家食品安全标准。
综合来看,施用钢渣可有效改良多金属复合污染土壤的性质,抑制水稻对重金属的吸收和转运,降低水稻特别是其地上部的重金属浓度。
关键词:土壤;重金属;钢渣;水稻;硅中图分类号:X53文献标志码:A文章编号:1672-2043(2011)03-0455-06Ameliorative Effects of Steel Slag Application on Multi-metal Contaminated Soil and Heavy Metal Uptake of RiceDENG Teng-hao-bo1,GU Hai-hong1,QIU Rong-liang1,2*(1.School of Environmental Science and Engineering,Sun Yat-sen University,Guangzhou510275,China;2.Guangdong Provincial Key Lab of Environmental Pollution Control and Remediation Technology,Guangdong510275,China.)Abstract:Heavy metal pollution of farmland soil is getting more and more serious in China nowadays due to mining activity,discharge of in-dustrial effluents and long-time application of pesticide and chemical fertilizers.Since heavy metals are toxic to crops,the continuous cultiva-tion in contaminated soil would decrease crop production capacity.Heavy metals can be also accumulated in human bodies through food chains.Application of soil amendments is an effective way to solve these kinds of problems,especially in slightly-and moderately-polluted soil.Steel slag is one kind of by-products of steel smelting.In this paper,pot experiment and field experiment were conducted to investigate the effects of steel slag application on pH value,available silicon and available heavy metal content(Cd,Pb,Cu,Zn)in multi-metal contami-nated soil and heavy metal uptake by rice plant.Five treatments were designed in the pot experiment consisting of CK(No slag),SS3and SS6(3g·kg-1and6g·kg-1of100mesh slag respectively),FSS3and FSS6(3g·kg-1and6g·kg-1of180mesh slag respectively).And the field ex-periment contained2treatment:CK(No slag)and SS(3g·kg-1of100mesh slag respectirely).The results of the pot experiment showed that soil pH and available silicon content increased with the increase of the amount of slag application and along with the decrease of the slag particle size.And soil available heavy metal content decreased significantly in the slag application treatments.Heavy metal concentration in shoot of rice plant decreased significantly with the slag application and was far lower than that in root.Heavy metal concentration in the root with the slag application sharply decreased in compared to CK.And the field experiment showed that steel slag application could dramatically increase rice production and decrease the heavy metal concentration in polished rice.In conclusion,steel slag application could effectively improve the quality of contaminated soil,restrain the heavy metal uptake of rice plant,and decrease the heavy metal concentration especially in the shoot of rice plant.Keywords:soil;heavy metal;steel slag;rice;silicon收稿日期:2010-08-18基金项目:国家高技术研究发展计划(863计划)重点项目(2007AA061001);国家自然科学基金-广东联合基金重点项目(U0833004)作者简介:邓腾灏博(1988—),男,广东始兴人,在读博士,主要从事土壤重金属污染修复研究。
橡胶初加工废水污染土壤的微生物固定化修复
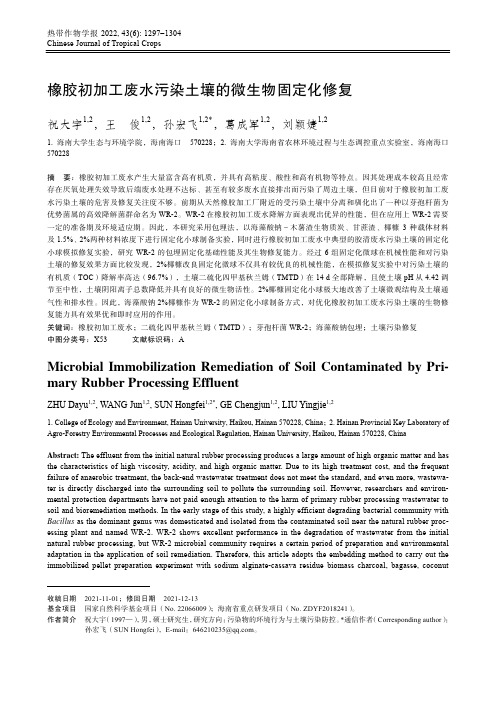
热带作物学报2022, 43(6): 1297 1304Chinese Journal of Tropical Crops橡胶初加工废水污染土壤的微生物固定化修复祝大宇1,2,王俊1,2,孙宏飞1,2*,葛成军1,2,刘颖婕1,21. 海南大学生态与环境学院,海南海口 570228;2. 海南大学海南省农林环境过程与生态调控重点实验室,海南海口570228摘要:橡胶初加工废水产生大量富含高有机质,并具有高粘度、酸性和高有机物等特点。
因其处理成本较高且经常存在厌氧处理失效导致后端废水处理不达标、甚至有较多废水直接排出而污染了周边土壤,但目前对于橡胶初加工废水污染土壤的危害及修复关注度不够。
前期从天然橡胶加工厂附近的受污染土壤中分离和驯化出了一种以芽孢杆菌为优势菌属的高效降解菌群命名为WR-2。
WR-2在橡胶初加工废水降解方面表现出优异的性能,但在应用上WR-2需要一定的准备期及环境适应期。
因此,本研究采用包埋法,以海藻酸钠-木薯渣生物质炭、甘蔗渣、椰糠3种载体材料及1.5%、2%两种材料浓度下进行固定化小球制备实验,同时进行橡胶初加工废水中典型的胶清废水污染土壤的固定化小球模拟修复实验,研究WR-2的包埋固定化基础性能及其生物修复能力。
经过6组固定化微球在机械性能和对污染土壤的修复效果方面比较发现,2%椰糠改良固定化微球不仅具有较优良的机械性能,在模拟修复实验中对污染土壤的有机质(TOC)降解率高达(96.7%),土壤二硫化四甲基秋兰姆(TMTD)在14 d全部降解,且使土壤pH从4.42调节至中性,土壤阴阳离子总数降低并具有良好的微生物活性。
2%椰糠固定化小球极大地改善了土壤微观结构及土壤通气性和排水性。
因此,海藻酸钠2%椰糠作为WR-2的固定化小球制备方式,对优化橡胶初加工废水污染土壤的生物修复能力具有效果优和即时应用的作用。
关键词:橡胶初加工废水;二硫化四甲基秋兰姆(TMTD);芽孢杆菌WR-2;海藻酸钠包埋;土壤污染修复中图分类号:X53 文献标识码:AMicrobial Immobilization Remediation of Soil Contaminated by Pri-mary Rubber Processing EffluentZHU Dayu1,2, WANG Jun1,2, SUN Hongfei1,2*, GE Chengjun1,2, LIU Yingjie1,21. College of Ecology and Environment, Hainan University, Haikou, Hainan 570228, China;2. Hainan Provincial Key Laboratory of Agro-Forestry Environmental Processes and Ecological Regulation, Hainan University, Haikou, Hainan 570228, ChinaAbstract: The effluent from the initial natural rubber processing produces a large amount of high organic matter and has the characteristics of high viscosity, acidity, and high organic matter. Due to its high treatment cost, and the frequent failure of anaerobic treatment, the back-end wastewater treatment does not meet the standard, and even more, wastewa-ter is directly discharged into the surrounding soil to pollute the surrounding soil. However, researchers and environ-mental protection departments have not paid enough attention to the harm of primary rubber processing wastewater to soil and bioremediation methods. In the early stage of this study, a highly efficient degrading bacterial community with Bacillus as the dominant genus was domesticated and isolated from the contaminated soil near the natural rubber proc-essing plant and named WR-2. WR-2 shows excellent performance in the degradation of wastewater from the initial natural rubber processing, but WR-2 microbial community requires a certain period of preparation and environmental adaptation in the application of soil remediation. Therefore, this article adopts the embedding method to carry out the immobilized pellet preparation experiment with sodium alginate-cassava residue biomass charcoal, bagasse, coconut收稿日期 2021-11-01;修回日期 2021-12-13基金项目 国家自然科学基金项目(No. 22066009);海南省重点研发项目(No. ZDYF2018241)。
气相抽提法去除土壤中的苯和乙苯
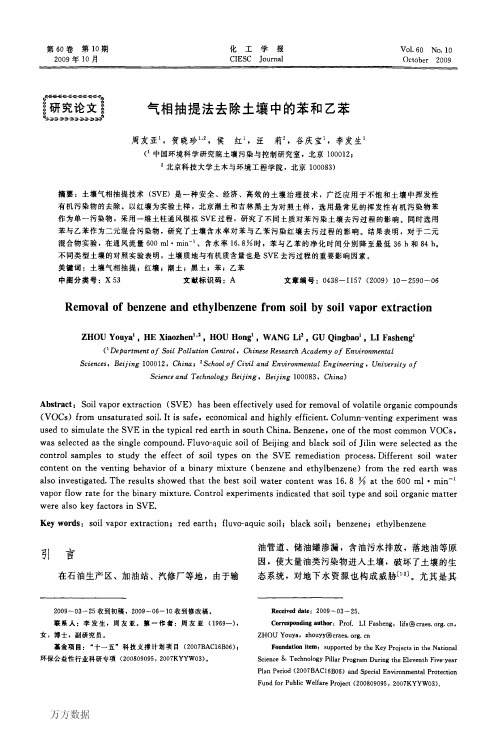
表l苯、乙苯理化性质
Table l
Physicochemi姐I properti龋0f benzene and ethyIbem伽【e
Sample
pH篙,筹。甓=2 B?颦:ic
/g。cm。 8.48 4.85 6.76 O.54 O.95 2.01 2.65 2.70 2.70 I.30 1.59 1.54
Reeeiw蚰date:2009一03—25.
Con惯poⅡding aⅡthor:Prof. LI Fasheng,lifs@craes.org.cn,
ZHOU Youya,zhouyy@craes.org.cn
Fo¨dati蚰it哪:
supported by the Key Projects
During
Vapor
was 1 6.8%at the 600 ml・min一1
flow
rate
for the binary mixture.Control experiments indicated that soil type and soil organic matter
were also kev factors in SVE. Key words: soil vapor extraction; red earth; fluvo—aquic soil; black soil; benzene; ethylbenzene
mm,膜厚O.5 pm),配氢火焰检测器
(FID)。
自行设计加工的,不同的是其他研究室一般采用单 柱,而本研究采用的是三组不锈钢柱,通过阀门控 制各个柱子既可独立也可同时使用。由空气压缩 机、过滤器、稳压罐、气体流量计、实验土柱、活 性炭柱和气体管道等组成(图1)。土柱采用9510×
零价纳米铁在靶场土壤修复中应用展望
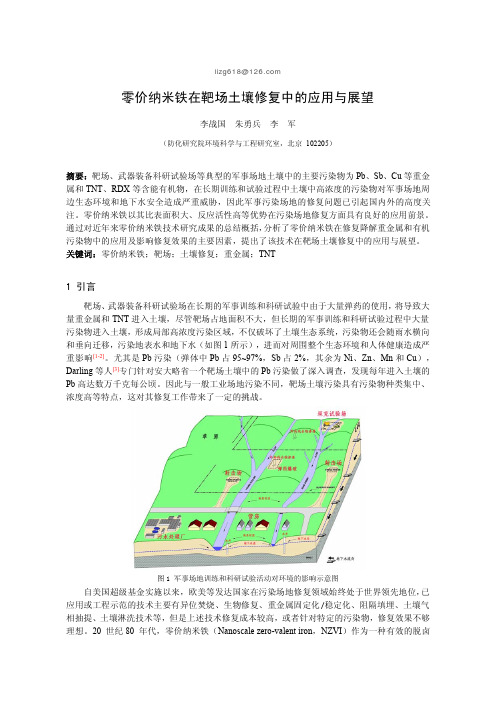
***************零价纳米铁在靶场土壤修复中的应用与展望李战国朱勇兵李军(防化研究院环境科学与工程研究室,北京102205)摘要:靶场、武器装备科研试验场等典型的军事场地土壤中的主要污染物为Pb、Sb、Cu等重金属和TNT、RDX等含能有机物,在长期训练和试验过程中土壤中高浓度的污染物对军事场地周边生态环境和地下水安全造成严重威胁,因此军事污染场地的修复问题已引起国内外的高度关注。
零价纳米铁以其比表面积大、反应活性高等优势在污染场地修复方面具有良好的应用前景。
通过对近年来零价纳米铁技术研究成果的总结概括,分析了零价纳米铁在修复降解重金属和有机污染物中的应用及影响修复效果的主要因素,提出了该技术在靶场土壤修复中的应用与展望。
关键词:零价纳米铁;靶场;土壤修复;重金属;TNT1引言靶场、武器装备科研试验场在长期的军事训练和科研试验中由于大量弹药的使用,将导致大量重金属和TNT进入土壤,尽管靶场占地面积不大,但长期的军事训练和科研试验过程中大量污染物进入土壤,形成局部高浓度污染区域,不仅破坏了土壤生态系统,污染物还会随雨水横向和垂向迁移,污染地表水和地下水(如图1所示),进而对周围整个生态环境和人体健康造成严重影响[1-2]。
尤其是Pb污染(弹体中Pb占95~97%,Sb占2%,其余为Ni、Zn、Mn和Cu),Darling等人[3]专门针对安大略省一个靶场土壤中的Pb污染做了深入调查,发现每年进入土壤的Pb高达数万千克每公顷。
因此与一般工业场地污染不同,靶场土壤污染具有污染物种类集中、浓度高等特点,这对其修复工作带来了一定的挑战。
图1军事场地训练和科研试验活动对环境的影响示意图自美国超级基金实施以来,欧美等发达国家在污染场地修复领域始终处于世界领先地位,已应用或工程示范的技术主要有异位焚烧、生物修复、重金属固定化/稳定化、阻隔填埋、土壤气相抽提、土壤淋洗技术等,但是上述技术修复成本较高,或者针对特定的污染物,修复效果不够理想。
重金属 污染土壤 生物蓄积性 耐受性

Journal of Environmental Sciences 20(2008)62–67Potential of Pteris vittata L.for phytoremediation of sites co-contaminated withcadmium and arsenic:The tolerance and accumulationXIAO Xiyuan,CHEN Tongbin ∗,AN Zhizhuang,LEI Mei,HUANG Zechun,LIAO Xiaoyong,LIU YingruCenter for Environmental Remediation,Institute of Geographic Sciences and Natural Resources Research,Chinese Academy of Sciences,Beijing 100101,China.E-mail:xiaoxy@Received 10April 2007;revised 21June 2007;accepted 11July 2007AbstractField investigation and greenhouse experiments were conducted to study the tolerance of Pteris vittata L.(Chinese brake)to cadmium (Cd)and its feasibility for remediating sites co-contaminated with Cd and arsenic (As).The results showed that P .vittata could survive in pot soils spiked with 80mg /kg of Cd and tolerated as great as 301mg /kg of total Cd and 26.8mg /kg of diethyltriaminepenta acetic acid (DTPA)-extractable Cd under field conditions.The highest concentration of Cd in fronds was 186mg /kg under a total soil concentration of 920mg As /kg and 98.6mg Cd /kg in the field,whereas just 2.6mg /kg under greenhouse conditions.Ecotypes of P .vittata were di fferentiated in tolerance and accumulation of Cd,and some of them could not only tolerate high concentrations of soil Cd,but also accumulated high concentrations of Cd in their fronds.Arsenic uptake and transportation by P .vittata was not inhibited at lower levels ( 20mg /kg)of Cd pared to the treatment without addition of Cd,the frond As concentration was increased by 103.8%at 20mg Cd /kg,with the highest level of 6434mg /kg.The results suggested that the Cd-tolerant ecotype of P .vittata extracted e ffectively As and Cd from the site co-contaminated with Cd and As,and might be used to remediate and revegetate this type of site.Key words :arsenic (As);cadmium (Cd);Chinese brake (Pteris vittata L.);hyperaccumulator;phytoremediation;tolerance;uptakeIntroductionLarge areas of land contaminated with Cd were caused by anthropogenic activities such as mining and mineral processing of metallic ores,waste disposal,phosphate fertilizer application and wastewater irrigation (McGrath et al .,2001;Udom et al .,2004;Lei et al .,2005).Soil Cd contamination is a great threat to human health since Cd is easily extracted by plants from the environment compared with other non-essential elements,and trans-ferred to human food chain from the soils (Lind´e n et al .,2003).Arsenic,also a highly toxic element,is a ubiquitous contaminant of global concern.As sites of As contamination often co-exists higher levels of other heavy metals such as Cd,Cu,Pb,Cr and Zn (Groudev et al .,2001;Kim et al .,2003),there is an increasing awareness of soils contaminated with As or co-contaminated with As and other metals (Loska et al .,2004;Liao et al .,2005)and the requirement for developing approaches for some remediation of the co-existing contaminants.Phytoremediation is emerging as a potential cost-e ffective solution for contaminated soils through the use of plants to remove pollutants (Salt et al .,1995).V´a zquez et al .(2006)studied the phytoextraction of Cd and As*Corresponding author.E-mail:chentb@.in Cd and As co-contaminated soils by lupin (Lupinus albus ),however,they did not recommend the plant for Cd and As phytoremediation due to its low removal,1.04g /(hm 2·a)for Cd and 8.06g /(hm 2·a)for As.Pteris vittata L.(Chinese brake),an As-hyperaccumulator discovered by Chen’s group (Chen and Wei,2000;Chen et al .,2002a)has the ability to hyperaccumulate As in its fronds.In ad-dition,it has characteristics of fast growing,high biomass production,wide geographic distribution and perennial.It has great potential for remediating As-contaminated sites (Chen et al .,2002b).A field phytoremediation of As-contaminated farmland using P .vittata has been suc-cessfully carried out in Chenzhou City,southern China since 2001,with the highest e fficiency of As removal being 7.84%after seven months of experiment (Liao et al .,2004).Our previous studies indicated that P .vittata had the potential to phytoremediate or revegetate soils co-contaminated with As and Zn (An et al .,2006).Fayiga et al .(2004)showed the capability of P .vittata in hyperac-cumulating As from soils was adversely impacted in the presence of Cd spiked with 50and 200mg /kg.Lei et al .(2005)found that the P .vittata growing in the Caishan tailing pond,Shizhuyuan mining areas could concentrate Cd in the roots up to 365mg /kg,which was 21.5times above the highest concentration of Cd in other fourteenj es c .ac .c nNo.1Potential of Pteris vittata L.for phytoremediation of sites co-contaminated with cadmium and arsenic (63)Table1Properties of the soil used in the greenhouse experiment Properties ValuepH7.9Organic matter content(g/kg)14.4Total N(g/kg) 1.1Olsen-P(mg/kg)19.0NH4OAc-extractable K(mg/kg)85.2Total Cd(mg/kg)0.08Total As(mg/kg) 5.5plant species investigated.Whether P.vittata can be used to remediate or revegetate mine tailings and soils co-contaminated with Cd and As depends on its Cd tolerance and accumulation.However,so far there is little informa-tion available on the tolerance and accumulation of Cd under As and Cd co-contaminated soil.Therefore,in this study,afield investigation and a greenhouse experiment were carried out to verify whether this plant has the potential to remediate sites polluted with Cd and As.1Materials and methods1.1Descriptions offield sitesBoth P.vittata and soil samples were collected from twenty-two sites in southern China where P.vittata is widely distributed.Sites1–7were located in Guangxi Province and Sites8–22in Hunan Province.Site1was located on farmland,Sites2and4in sewage ditches,Site 3on a domestic garbage dump,Site5on farmland near a Mn mine area,Site7on a sunken riverbed,and Sites6and 8–22around mining,metal refinery or tailing areas.1.2Greenhouse experimentSpores of P.vittata collected from arsenic mining areas in Shimen County,Hunan Province were scattered onto a moist soil in a seedbed covered with a plastic clingfilm to maintain moisture.After the spores germinated and grew into sporelings with true leaves,the plants were watered and fertilized as needed.The soil used for cultivation was a loam cinnamon soil(Typical Agric-Udic-Luvisols)collected from the top layer(0–20cm)of farmland in Beijing,China,then air-dried and passed through a2-mm sieve for the greenhouse experiment.Soil pH was measured using a pH meter in a ratio of soil:solution=1:2.5(w/v).Important properties of the soil determined according to the methods described by Page et al.(1982)are listed in Table1.Basal fertilizers of0.4gN/kg as(NH4)2SO4,0.2gP/kg and0.25gK/kg as KH2PO4,and400mg As/kg as Na2HAsO4·7H2O were mixed with the soil,and then0.5kg of the soil was placed into each plastic pot.Cadmium as CdCl2was added to the pots at concentrations of0,10,20,60,80mg/kg, respectively.Each treatment was replicated four times. After an equilibration time of1month,4plants with3or4 fronds were transplanted into each pot,and were grown in a greenhouse with temperatures between22°C(night)and 30°C(day),with a12-h light period.Afterfifteen weeks of growth,the plants were harvested.1.3Sampling and analysisP.vittata grown in the greenhouse experiment and col-lected from the sites studied were separated into rhizoidsand fronds.They were washed with tap water,and thenrinsed with deionized water.Plant samples were dried at60°C for48h,and weighed.Topsoil samples(0–20cm)from thefield in which the plants were growing werealso collected,air-dried,ground using an agate mortar,sieved through a2-mm screen,then pulverized and passed through a sieve of0.149mm for analysis.The soils were digested with HNO3-H2O2(Chen etal.,2002a),and the plants were digested with HNO3-HClO4(Liao et al.,2004).Diethyltriaminepenta aceticacid(DTPA)-extractable Cd was extracted by0.005mol/L diethylenetriamine pentaacetic acid,0.01mol/L CaCl2and0.1mol/L triethanolamine buffered at pH7.3(Bailey etal.,1995).Cadmium was determined by a graphite furnace atomic absorption spectrophotometer(Vario6,Analytic Jena,German),and As was analyzed using a hydrogen generation-atomicfluorescence spectrometer(HG-AFS)(AFS-2202,Beijing Haiguang Instrumental Co.,China).Blank and standard reference materials for plant(GBW-07603)and soil(GBW-07401)samples obtained from theChina National Center for Standard Reference Materialswere included for the Quality Assurance/Quality Control program.1.4Data analysisData were statistically performed with one-way ANOV Ausing SPSS13.0for Windows,and means among treat-ments for a given property were subjected to LSD test at P<0.05.2Results2.1Soil Cd concentration at sampling sitesGreat variations in Cd concentrations were found in thesoils from the investigated sites,ranging from0.17to301mg/kg(Table2).Most of the total soil Cd concentrationsat the sampling sites were higher than the backgroundsoil Cd concentrations(arithmetic means)of0.27mg/kgin Guangxi Province and0.13mg/kg in Hunan Province,China(CNEMC,1990),which indicated slight or great elevations of soil Cd levels at these sites.The soil As concentrations varied in the range of7.6–32.2mg/kg atmost of the sites that were far away from polluted areas. However,for Sites11–22,high As concentrations usually occurred together with high Cd concentrations in soilsfrom the same site(Table2).These results indicated that P.vittata could grow well at sites seriously co-contaminatedwith As and Cd,indicating its capability for revegetationof sites co-contaminated with As and Cd.The DTPA-extractable soil Cd was positively correlatedwith the total soil Cd(P<0.05).The maximum concen-tration of DTPA-extractable Cd was26.8mg/kg at Site15,and the value at Site22was13.1mg/kg and this accountedfor13.2%of the total soil Cd.The soil pH of the sitesvaried from4.6at Site17to8.4at Site19,indicating thatjesc.ac.cn64XIAO Xiyuan et al.V ol.20Table 2Soil pH and concentrations of Cd and As in soils and Pteris vittata of di fferent field sitesSite No.pH Soil As Soil Cd (mg /kg)Plant As (mg /kg)Plant Cd (mg /kg)Cd (mg /kg)Total DTPA-Cd Rhizoid Frond Rhizoid Frond BF TF17.615.4 1.420.70ND 6.53ND 0.060.0428.023.20.540.09ND 150ND 0.070.1337.625.30.490.07 6.7852.90.030.080.17 2.547.720.40.30ND 29.52100.140.220.74 1.557.97.6 2.430.2511594.70.100.130.05 1.36 6.721.9 1.130.0931.32120.110.110.1 1.07 5.213.90.170.06ND 90.8ND 0.010.0387.619.3 3.060.143632570.120.120.04 1.0297.88.8 1.710.05225269 3.87 3.31 1.90.86107.415.7 1.520.187.8527.1 3.80 3.74 2.50.98117.0154725.4 4.181074617 4.61 4.690.18 1.0127.39406 4.81 2.7784538813.38.02 1.70.60137.23130.900.437851123 3.04 3.45 3.8 1.1148.0 5.5 5.470.181033683 4.00 4.810.88 1.20157.832.230126.819.4157226320.10.070.08168.157210.7 1.231068294ND 11.4 1.114.817 4.6911843.8 2.0297.12362 2.27 2.930.07 1.318 6.6146622.3 1.2220672680 3.61 5.330.24 1.5198.493217.20.58726304 6.8315.30.89 2.320 6.230.0 6.970.9135.028.0 6.4310.8 1.5 1.7218.1694122 5.1213.587.219.616.50.140.84227.192098.613.1193093.31601861.91.2ND:not detected;BF (bioconcentration factor)means the ratio of the Cd concentration in fronds of P .vittata to that in soil;TF (translocation factor)stands for the ratio of the Cd concentration in fronds to that in rhizoids of P .vittata .most of the soils were alkaline (Table 2).2.2Cd uptake and transportation by P .vittata in the field Cadmium concentrations in P .vittata grown at field sites varied substantially with changes in the soil Cd concentrations (Table 2).There was a significant positive correlation between soil DTPA-extractable Cd and the rhizoid Cd concentration (P <0.05).Both rhizoid and frond Cd concentrations of the plants at Sites 1–8with lower soil Cd concentrations were less than 1.0mg /kg,and most of the rhizoid Cd concentrations of the plants grown at mining or refinery areas were more than3.0mg /kg,while the frond Cd concentrations at some sites were approximately 10.0mg /kg.The maximum rhizoid Cd concentration was 263mg /kg at Site 15,and the frond Cd concentration reached 186mg /kg at Site 22(Table 2).On the other hand,P .vittata grew well in the soil with 9406mg /kg of As,and had high capacity to accumulate As in the frond with the highest As level of 2680mg /kg.Bioconcentration factors (BFs)for Cd in P .vittata grown at Sites 1–8were less than 1.0(Table 2).There were also low BFs for Cd at severely contaminated sites,i.e.Sites 17and 21.However,soil Cd could be e ffectively accumulated by P .vittata at Sites 9,10,13and 20,where the BFs were greater than 1.0,and the maximum value was as high as 3.8.P .vittata also e ffectively transport Cd from rhizoids to fronds.The translocation factors (TFs)for Cd at most of the sites were close to 1.0.The BF and TF at the heavily Cd-contaminated Site 22were 1.9and 1.2,respectively (Table 2).2.3Cadmium accumulation of P .vittata in the green-house Addition of Cd to the soil under greenhouse conditionsFig.1Biomass of P .vittata after transplanting for 15weeks in soils spiked with 400mg /kg of As and various concentrations of Cd.Values are presented as means ±standard deviations for 4replicates.obviously inhibited the growth of P .vittata ,and its biomass sharply declined in pot experiment (Fig.1).Compared to the treatment without Cd addition,the dry weights of rhizoid,frond and total biomass were decreased by 77.9%,69.7%and 71.8%,respectively in the treatment with the addition of 10mg Cd /kg (Fig.1).These values of the other treatments with Cd addition were also lower than those of the control (Fig.1).The results show that all the treatments with di fferent concentrations of Cd added to soil in this experiment had a strong toxicity to the sporelings of P .vittata .The addition of Cd to pot soil in the greenhouse ex-periments could promote Cd uptake by P .vittata .Rhizoid and frond Cd concentrations were 3.76and 0.37mg /kg in the control,and increased to 7.35and 1.37mg /kg in the treatment with the addition of 10mg Cd /kg (Fig.2a).Rhizoid Cd concentrations were significantly enhanced with increase of added Cd concentrations over the range of 10–20mg /kg,while the di fference in frond Cd con-j es c .ac .c nNo.1Potential of Pteris vittata L.for phytoremediation of sites co-contaminated with cadmium and arsenic······65Fig.2Cd concentrations(a)and accumulations(b)in P.vittata after transplanting for15weeks in soils spiked with400mg/kg of As and various concentrations of Cd.Values are means±standard deviations for4replicates.centrations is not significant(P>0.05).Rhizoid and frond Cd concentrations increased to61.2and2.63mg/kg, respectively when the concentration of Cd added was 60mg/kg,and then decreased with the addition of80 mg Cd/kg(Fig.2a).Cadmium taken up by the plant was predominantly retained in the rhizoid,and the TF of Cd was reduced with increasing concentrations of added Cd. The TF was0.19for the treatment of addition10mg Cd/kg and decreased to0.04with60mg Cd/kg.The Cd accumulations(Cd concentration×biomass)in P.vittata shifted with the added Cd concentrations.The plant in the soil added with20mg Cd/kg showed lower Cd accumulation than the other Cd-spiked soils,while the maximum frond and rhizoid Cd accumulations were2.47 and51.0µg/pot,respectively for the treatment with the addition of60mg Cd/kg(Fig.2b).2.4Effects of Cd on As accumulation by plants undergreenhouse conditionAs compared to the control,rhizoid As concentrations increased by48.0%and23.0%for the treatments with the additions of60and80mg Cd/kg,respectively(Fig.3a),and frond As concentrations were increased remarkably with the addition of lower concentrations of Cd( 20mg/kg); these were enhanced38.5%and103.8%in the soils spiked with10and20mg Cd/kg,respectively,reaching to6434 mg/kg at20mg Cd/kg.However,for the treatments with higher concentrations of Cd addition( 60mg/kg),frond As concentrations decreased by25.6%at80mg Cd/kg in comparison to the control(Fig.3a).The TF of As in the treatments spiked with lower Cd concentrations( 20mg/kg)was much greater than that of the control(Fig.3b).The TF was75.4%higher at10 mg Cd/kg than the control,and decreased visibly with higher concentrations of Cd applied to the soil,suggesting that application of lower Cd concentrations favored the transportation of As from rhizoid to frond.Although addition of Cd to soil might disturb metabolisms in P. vittata,As uptake and transportation was not inhibited by lower addition concentrations of Cd.The amount of As accumulated in fronds of P.vittata reduced markedly due to the decrease in its biomass for all the treatments spiked with various Cd concentrations. The As accumulation of P.vittata was much higher in the control than those in the Cd-amended soils,and decreasedby57.8%and82.3%in the treatments with addition of10and80mg Cd/kg,respectively(Fig.3b),showing that theefficiency of As accumulation in P.vittata was adverselyaffected by Cd.3DiscussionThefield investigation showed that P.vittata grew wellat manyfield sites where the total Cd concentration insoil was much higher than10mg/kg,greatly exceed-ing the ranges which were considered toxic to normalplants(Dong and Chen,1982).Cd-hyperaccumulatingplant species such as Brassicaceae(Thlaspi caerulescens), amongst higher plants,are almost the only ones that cangrow in soil solutions containing Cd concentrations ashigh as35µmol/L(3.9mg/L)(Brown et al.,1994).Novisible symptoms of Cd toxicity in P.vittata atfield Sites11,15,21and22heavily contaminated with Cd,wherethe highest total soil concentrations of Cd and DTPA-extractable Cd were301and26.8mg/kg(238µmol/L), respectively(Table2),representing1115times of their soil background Cd levels for Guangxi and2315times for Hu-nan(CNEMC,1990).Out of the22sites investigated,soilCd concentrations at12sites were higher than3mg/kg of Australia Ecological Investigation Levels for Soils(NEPC, 1999).Furthermore,the pot experiment showed that thisplant survived in the soil added with80mg Cd/kg,thoughits growth was inhibited.Our previousfield survey also showed that P.vittata grew well at contaminated siteswith high level of Cd(32.1mg/kg)(Lei et al.,2005).Sothis plant growing in the polluted site exhibited strong Cd adaptability.There were significant variations in Cd uptake and transportation for populations of P.vittata.Concentrationsof Cd in the fronds of this plant grown at Sites11,15,21and22were4.69,20.1,16.5and186mg/kg, respectively with corresponding TFs of Cd of1.02,0.08,0.84and1.16(Table2).Cadmium has strong toxicityto plants,and the normal range of Cd concentration inleaf tissues(dry weight)of some plant species is ap-proximate0.05–0.2mg/kg,and the excessive or toxic concentrations are5–10up to30mg/kg(Kabata-Pendiasand Pendias,2001).The frond Cd concentrations injesc.ac.cn66XIAO Xiyuan et al.V ol.20Fig.3As concentrations(a)and accumulations(b)in P.vittata after transplanting for15weeks in pot soils spiked with400mg/kg of As and various concentrations of Cd.Values are means±standard deviations for4replicates.the plants collected from many sites investigated were higher than5mg/kg(Table2),showing that P.vittata had exceptional ability to tolerate and accumulate Cd in the fronds.Heavy metals act as a powerful selective force for the evolution of tolerant populations of plants (McNeilly and Bradshaw,1968).Meerts and Van Iascker (1997)reported that non-metallicolous populations(T. caerulescens Subsp.T.caerulescens)from the Crand-Duchy of Luxembourg have a lower tolerance and a higher zinc accumulation capacity compared with metallicolous population(T.caerulescens Subsp.calaminare)of Prayon and Plombi`e res in Belgium.Escarr´e et al.(2000)also showed a higher Cd hyperaccumulation capacity for the metallicolous ecotype compared to the non-metallicolous populations from southern France.Therefore,populations of P.vittata growing at Cd-contaminated sites might evolve into Cd-tolerant ecotypes.However,addition of 10mg Cd/kg under greenhouse conditions with DTPA-extractable Cd of4.3mg/kg inhibited the growth of P. vittata.(Fig.1),and the frond Cd concentration was only 2.6mg/kg,with a low BF and TF for addition of60mg Cd/kg(Fig.2).However,Fayiga et al.(2004)reported that the total biomass of P.vittata growing in the soil spiked with50mg/kg of Cd increased by125%compared to the treatment without Cd addition in greenhouse experiments. They speculated that the stimulation of plant growth by Cd might result from added N nutrition since Cd was added as nitrate salts.In our previous study,however,there were no significant differences of biomass of P.vittata between treatments spiked with0.1mol Ca/L in the form of Ca(NO3)2and those applied with Ca as CaCl2in solution under sand culture(Liao et al.,2003).It was implied that the plants propagated from spores collected from sites with low Cd concentrations such as As mine areas had less tolerance to Cd than those grown on Cd-contaminated sites.The results from the greenhouse experiment and field investigation suggest that ecotypical differences in Cd tolerances of P.vittata to Cd be present.At some sites heavily contaminated with As,i.e.Sites 13,15,17and18,the As concentrations in the frond were far above1000mg/kg,with the highest As concentration of2680mg/kg under total soil As level of1466mg/kg (Table2),which also support the demonstration that this plant has high capacity to concentrate As in the fronds (Chen and Wei,2000;Chen et al.,2002a).In addition,the greenhouse experiment results showed that addition oflow Cd concentrations( 20mg Cd/kg)to soil increasedthe frond As concentration.Thefield investigation results indicated that some ecotypes of P.vittata tolerated high concentrations of soil Cd and As;on the other hand,P.vittata grown at Site22could hyperaccumulate Cd(186mg/kg)in its frond,exceeding the standard of100mg/kgfor a Cd-hyperaccumulator(Baker et al.,1994;Brookset al.,1998).P.vittata can grow up to2m height with biomass production reaching as high as36t/hm2(fresh weight)under the favorable environmental conditions inthefield(Chen et al.,2002a),which has the greater harvestable biomass compared to most documented Cd hyperaccumulators such as T.caerulescens with only small aboveground biomass(Cobbett and Meagher,2002).These results imply that some ecotypes of P.vittata offer a great potential for phytoremediation of Cd contaminated soils. Hence,it is feasible to select Cd-tolerant ecotypes of P.vittata to phytoremediate and revegetate As and Cd co-contaminated sites.Our previous study demonstrated thatthis plant could not only tolerate high As but also highZn levels in growing soils.It grew healthily in the soil applied with1000mg/kg of Zn(An et al.,2006).Thehigh tolerance of P.vittata to Cd and the other heavy metalis important characteristics required for phytoremediationof multiple-metal contaminated soils.The mechanism forthe co-tolerance of P.vittata to Cd and the other metal toxicities remains to be studied in the future.4ConclusionsThe present results from afield investigation and greenhouse experiments results show great ecotypical dif-ferences in the tolerance of P.vittata to Cd.Some of the ecotypes had a high tolerance to Cd,which could growunder very high level of soil Cd(301mg/kg)and As (9118mg/kg).The plants grown at As and Cd co-pollutedsoils effectively absorbed and transported Cd from soil,with a frond Cd concentration of186mg/kg and a TF of1.2.The pot experiment showed that P.vittata also could survive in the soil added with80mg/kg of Cd.Further-more,addition of lower concentrations of Cd obviously increased the frond As concentrations.Therefore,a Cd-jesc.ac.cnNo.1Potential of Pteris vittata L.for phytoremediation of sites co-contaminated with cadmium and arsenic (67)tolerant ecotype of P.vittata could be used to remediate sites co-contaminated with Cd and As. AcknowledgementsThis work was supported by the National Science Foun-dation for the Distinguished Young Scholar of China(No. 40325003).ReferencesAn Z Z,Huang Z C,Lei M,Liao X Y,Zheng Y M,Chen T B,2006.Zinc tolerance and accumulation in Pteris vittata L.and its potential for phytoremediation of Zn-and As-contaminated soil.Chemosphere,62(5):796–802.Bailey V L,Grant C A,Racz G J,Bailey L D,1995.A practical method for assessing cadmium levels in soil using DTPA extraction technique with graphite furnace analysis.Commun Soil Sci Plant Anal,26:961–968.Baker A J M,Reeves R D,Hajar A S M,1994.Heavy metal accumulation and tolerance in British populations of the metallophyte Thlaspi caerulescens J.&C.Presl(Brassi-caceae).New Phytol,127:61–68.Brooks R R,Chambers M F,Nicks L J,Robinson B H,1998.Phytomining.Trends Plant Sci,3:359–362.Brown S L,Chaney R L,Angle J S,Baker A J M,1994.Phy-toremediation potential of Thlaspi caerulescens and bladder campion for zinc-and cadmium-contaminated soils.J Env-iron Qual,23:1151–1157.Chen T B,Wei C Y,2000.Arsenic hyperaccumulation in some plant species in South China.Proceedings of International Conference of Soil Remediation.Hangzhou,China,194–195.Chen T B,Wei C Y,Huang Z C,Huang Q F,Lu Q G,Fan Z L, 2002a.Arsenic hyperaccumulator Pteris vittata L.and its arsenic accumulation.Chin Sci Bull,47(11):902–905. Chen T B,Fan Z L,Lei M,Huang Z C,Wei C Y,2002b.Effect of phosphorus on arsenic accumulation in As-hyperaccumulator Pteris vittata L.and its implication.Chin Sci Bull,47(22):1876–1879.CNEMC(China National Environmental Monitoring Center), 1990.The background concentrations of soil elements in China.Beijing:China Environmental Science Press.334–335.Cobbett C S,Meagher R B,2002.Arabidopsis and the genetic potential for the phytoremediation of toxic elements and organic pollutants.In:The Arabidopsis Book(SomervilleC.,Meyerowitz,E.,eds.).USA:American Society of PlantBiologists,1543–8120.Dong K Y,Chen J M,1982.The relationship between the growth and cadmium uptake in the crops affected by cadmium.Environ Sci,3(4):31–34.Escarr´e J,Lefe`e bvre C,Gruber W,Leblanc M,Lepart J,Rivi`e re Y,Delay B,2000.Zinc and cadmium hyperaccumulation by Thlaspi caerulescens from metalliferous and nonmetal-liferous sites in the Mediterranean area:implications for phytoremediation.New Phytol,145:429–437.Fayiga A O,Ma L Q,Cao X,Rathinasabapathi B,2004.Effects of heavy metals on growth and arsenic accumulation in the arsenic hyperaccumulator Pteris vittata L.Environ Pollut, 132:289–296.Groudev S N,Spasova I I,Georgiev P S,2001.In situ bioreme-diation of soils contaminated with radioactive elements andtoxic heavy metals.Int J Miner Process,62:301–308.Kabata-Pendias A,Pendias H,2001.Trace elements in soils and plants.3rd ed.Boca Raton,Florida:CRC Press.Kim,M J,Ahn K H,Jung Y,Lee S,Lim B R,2003.Arsenic, cadmium,chromium,copper,lead,and zinc contaminationin mine tailings and nearby streams of three abandonedmines from Korea.Bull Environ Contam Toxicol,70(5):942–947.Lei M,Yue Q L,Chen T B,Huang Z C,Liao X Y,Liu Y R,ZhengG D,Chang Q R,2005.Heavy metal concentrations insoils and plants around Shizhuyuan Mining Area of HunanProvince.Acta Ecologica Sinica,25(5):1146–1151.Liao X Y,Xiao X Y,Chen T B,2003.Effects of Ca and As addition on As,P and Ca uptake by hyperaccumulatorPteris vittata L.under sand culture.Acta Ecologica Sinica,23(10):2057–2085.Liao X Y,Chen T B,Xie H,Xiao X Y,2004.Effect of application of P fertilizer on efficiency of As removal in contaminatedsoil using phytoremediation:Field demonstration.ActaScientiae Circumstantiae,24(3):455–462.Liao X Y,Chen T B,Xie H,Liu Y R,2005.Soil As contamination and its risk assessment in areas near the industrial districtsof Chenzhou City,Southern China.Environ Int,31:791–798.Lind´e n A,Olsson I M,Bensryd I,Lundh T,Skerfving S,Os-karsson A,2003.Monitoring of cadmium in the chain fromsoil via crops and feed to pig blood and kidney.EcotoxicolEnviron Saf,55(2):213–222.Loska K,Wiechula D,Korus I,2004.Metal contamination of farming soils affected by industry.Environ Int,30:159–165. McGrath S P,Zhao F J,Lombi E,2001.Plant and rhizo-sphere processes involved in phytoremediation of metal-contaminated soils.Plant Soil,232:207–214.McNeilly T,Bradshaw A D,1968.Evolutionary processes in populations of copper tolerant Agrostis tenuis.Evolution,22:108–118.Meerts P,Van Isacker N,1997.Heavy metal tolerance and accumulation in metallicolous and non-metallicolous pop-ulations of Thlaspi caerulescens from continental Europe.Plant Ecol,133:221–231.NEPC(National Environmental Protection Council),1999.Schedule B(1)Guideline on the Investigation Levels forSoil and Groundwater.National Environmental Protection(Assessment of Site Contamination).Canberra.Page A L,Miller R H,Keeney D R,1982.Methods of Soil Analysis.Madison,Wisc,USA:ASA-SSSA Inc.Salt D E,Blaylock M,Kumar N P,Dushenkov V,Ensley B D, Chet I,Raskin I,1995.Phytoremediation:a novel strategyfor the removal of toxic metals from the environment usingplants.Biotechnology,13:468–474.Udom B E,Mbagwu J S C,Adesodun J K,Agbim N N, 2004.Distributions of zinc,copper,cadmium and lead ina tropical ultisol after long-term disposal of sewage sludge.Environ Int,30(4):467–470.V´a zquez S,Agha R,Granado A,Sarro M J,Esteban E,Pealosa J M,Carpena R O,e of white lupin plant forphytostabilization of Cd and As polluted acid soil.WaterAir Soil Pollut,177:349–365.jesc.ac.cn。
黑麦草-根内球囊霉联合修复铀污染土壤
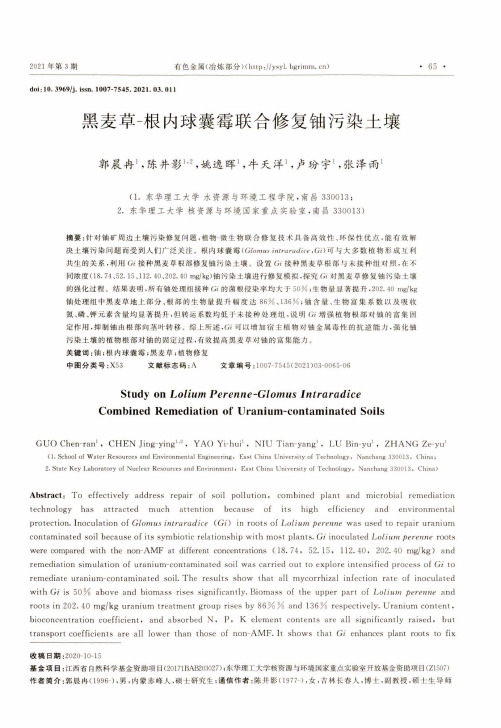
2021年第3期有色金属(冶炼部分)(http://y sy l. bgrimm. cn)• 65 •doi: 10. 3969/j. issn. 1007-7545. 2021. 03. Oil黑麦草-根内球囊霉联合修复铀污染土壤郭晨冉1,陈井影“2,姚逸晖1,牛天洋1,卢玢宇1,张泽雨1(1•东华理工大学水资源与环境工程学院,南昌330013;2.东华理工大学核资源与环境国家重点实验室,南昌330013)摘要:针对铀矿周边土壤污染修复问题,植物-微生物联合修复技术具备高效性、环保性优点,能有效解决土壤污染问题而受到人们广泛关注。
根内球囊霉可与大多数植物形成互利共生的关系,利用接种黑麦草根部修复铀污染土壤。
设置G/接种黑麦草根部与未接种组对照,在不同浓度(18. 74、52. 15、112. 40、202. 40 mg/kg)铀污染土壤进行修复模拟,探究G/对黑麦草修复铀污染土壤的强化过程。
结果表明,所有铀处理组接种G/的菌根侵染率均大于50%;生物量显著提升,202. 40 mg/kg铀处理组中黑麦草地上部分、根部的生物量提升幅度达86%、136%;铀含量、生物富集系数以及吸收氮、磷、钾元素含量均显著提升,但转运系数均低于未接种处理组.说明G z增强植物根部对铀的富集固定作用,抑制铀由根部向茎叶转移。
综上所述,G可以增加宿主植物对铀金属毒性的抗逆能力,强化铀污染土壤的植物根部对铀的固定过程,有效提高黑麦草对铀的富集能力。
关键词:铀;根内球囊霉;黑麦草;植物修复中图分类号:X53 文献标志码:A文章编号:1007-7545(2021)03-0065-06Study on L o l i u m P e r e n n e-G l o m u s I n t r a r a d i c eCombined Remediation of Uranium-contaminated SoilsGUO Chen-ran1 ,CHEN Jing-ying1,2,YAO Yi-hui1 ,NIU Tian-yang1 ,LU Bin-yu1 ,ZHANG Ze-yu1(1. School of W ater Resources and Environm ental Engineering, East China U niversity of Technology, Nanchang 330013, C hina;2. State Key Laboratory of Nuclear Resources and Environm ent, East China U niversity of Technology, Nanchang 330013, China)Abstract:To effectively address repair of soil pollution,combined plant and microbial remediation technology has attracted much attention because of its high efficiency and environmental protection.Inoculation of Glomus intraradice(G i)in roots of Lolium perenne was used to repair uranium contaminated soil because of its symbiotic relationship with most plants.Gi inoculated Lolium perenne roots were compared with the non-AMF at different concentrations(18.74,52.15,112. 40, 202.40 mg/kg)and remediation simulation of uranium-contaminated soil was carried out to explore intensified process of Gi to remediate uranium-contaminated soil.The results show that all mycorrhizal infection rate of inoculated with Gi is 50%above and biomass rises significantly.Biomass of the upper part of Lolium perenne and roots in 202. 40 mg/kg uranium treatment group rises by 86%%and 136%respectively.Uranium content, bioconcentration coefficient,and absorbed N,P,K element contents are all significantly raised,but transport coefficients are all lower than those of non-AMF.It shows that Gi enhances plant roots to fix收稿日期=2020-10-15基金项目:江西省自然科学基金资助项目(20171BAB203027);东华理工大学核资源与环境国家重点实验室开放基金资助项目(Z1507) 作者简介:郭晨冉(1996-),男,内蒙赤峰人,硕士研究生;通信作者:陈井影(1977-),女,吉林长春人,博士 •副教授,硕士生导师• 66 •有色金属(冶炼部分)(http://ysyl. bgrimm. cn}2021年第3期uranium accumulation,and can inhibit uranium transfer from roots to stems and leaves.In summary,Gi can raise resistance of host plants to uranium metal toxicity,strengthen fixation process of uranium by plant roots in uranium-contaminated soil,and can effectively improve uranium accumulation ability of Lolium perenne. Keywords:uranium;Glomus ijitraradice (G i);Lolium perenne;phytoremediation铀冶金工业产生的废渣引起放射性核素污染已 成为当今全球广泛关注的环境问题131。
土壤污染的危害英语作文
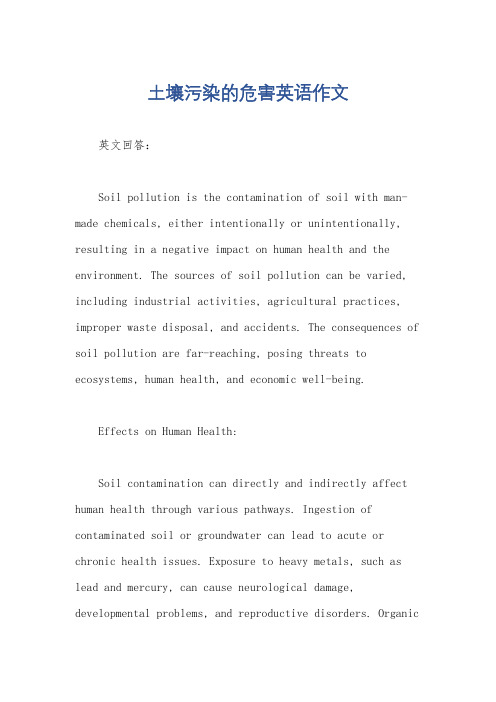
土壤污染的危害英语作文英文回答:Soil pollution is the contamination of soil with man-made chemicals, either intentionally or unintentionally, resulting in a negative impact on human health and the environment. The sources of soil pollution can be varied, including industrial activities, agricultural practices, improper waste disposal, and accidents. The consequences of soil pollution are far-reaching, posing threats to ecosystems, human health, and economic well-being.Effects on Human Health:Soil contamination can directly and indirectly affect human health through various pathways. Ingestion of contaminated soil or groundwater can lead to acute or chronic health issues. Exposure to heavy metals, such as lead and mercury, can cause neurological damage, developmental problems, and reproductive disorders. Organicpollutants, like polycyclic aromatic hydrocarbons (PAHs)and pesticides, have been linked to an increased risk of cancer, respiratory diseases, and immune system dysfunction.Impact on Ecosystems:Soil pollution disrupts the delicate balance of ecosystems. The accumulation of toxic substances in soilcan alter the soil chemistry, making it less hospitable for plant growth. Contaminated soil impairs nutrient uptake and water retention, leading to reduced plant productivity and stunted vegetation. The loss of soil biodiversity,including microorganisms and invertebrates, further degrades ecosystem resilience and ecosystem services.Economic Consequences:Soil pollution can have significant economic repercussions. Contaminated soil reduces land use for agricultural purposes, leading to decreased crop yields and lower-quality produce. The cleanup and remediation of polluted sites involve substantial costs, both forgovernments and private entities. The loss of ecosystem services, such as water filtration and carbon sequestration, also translates into economic losses.Mitigation and Prevention:Addressing soil pollution requires a multifaceted approach. Sustainable agricultural practices, such as reducing the use of chemical fertilizers and pesticides,can minimize soil contamination from agriculture. Proper waste management, including responsible disposal ofindustrial and household waste, is crucial in preventingsoil pollution. Implementing land-use planning strategiesto protect sensitive areas and prevent sprawl can help preserve uncontaminated soil resources.In summary, soil pollution poses a serious threat to human health, ecosystems, and the economy. Its effects are far-reaching and require urgent action. By implementing sustainable practices, promoting waste management, and protecting uncontaminated areas, we can mitigate soil pollution and ensure the health of our environment forfuture generations.中文回答:土壤污染的危害。
土地污染的结果英语版作文
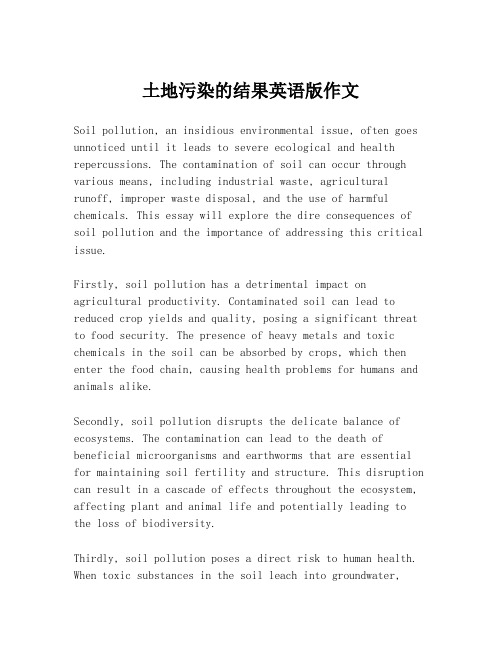
土地污染的结果英语版作文Soil pollution, an insidious environmental issue, often goes unnoticed until it leads to severe ecological and health repercussions. The contamination of soil can occur through various means, including industrial waste, agricultural runoff, improper waste disposal, and the use of harmful chemicals. This essay will explore the dire consequences of soil pollution and the importance of addressing this critical issue.Firstly, soil pollution has a detrimental impact on agricultural productivity. Contaminated soil can lead to reduced crop yields and quality, posing a significant threat to food security. The presence of heavy metals and toxic chemicals in the soil can be absorbed by crops, which then enter the food chain, causing health problems for humans and animals alike.Secondly, soil pollution disrupts the delicate balance of ecosystems. The contamination can lead to the death of beneficial microorganisms and earthworms that are essential for maintaining soil fertility and structure. This disruption can result in a cascade of effects throughout the ecosystem, affecting plant and animal life and potentially leading to the loss of biodiversity.Thirdly, soil pollution poses a direct risk to human health. When toxic substances in the soil leach into groundwater,they can contaminate drinking water supplies. Long-term exposure to these contaminants can lead to a range of health issues, including neurological disorders, organ damage, and increased risk of certain cancers.Moreover, soil pollution can also contribute to climate change. Polluted soils release greenhouse gases such as methane and carbon dioxide when they degrade organic matter. This release exacerbates global warming and leads to further environmental degradation.Addressing soil pollution requires a multifaceted approach. It involves implementing stricter regulations on industrial waste disposal, promoting sustainable agricultural practices, and raising public awareness about the importance of soil conservation. Additionally, the remediation of contaminated sites is crucial to restore the health of the soil and prevent further harm to the environment and human health.In conclusion, soil pollution is a pressing environmental challenge with far-reaching consequences. It affects agricultural productivity, disrupts ecosystems, endangers human health, and contributes to climate change. It is imperative that we take collective action to mitigate this issue and preserve our soil for future generations.。
土壤污染给人类带来的后果短篇英文作文
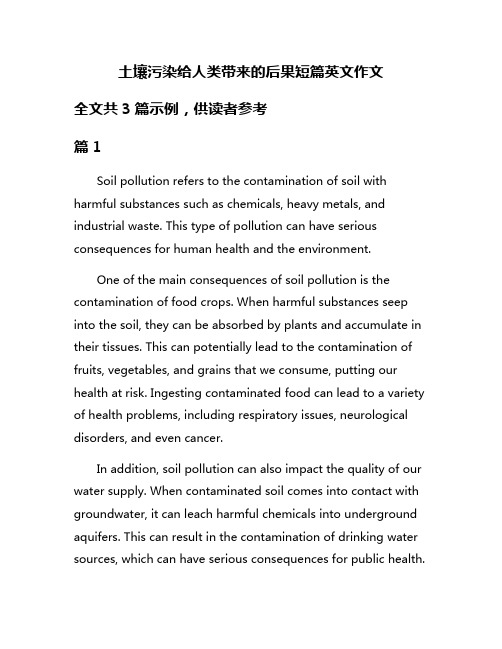
土壤污染给人类带来的后果短篇英文作文全文共3篇示例,供读者参考篇1Soil pollution refers to the contamination of soil with harmful substances such as chemicals, heavy metals, and industrial waste. This type of pollution can have serious consequences for human health and the environment.One of the main consequences of soil pollution is the contamination of food crops. When harmful substances seep into the soil, they can be absorbed by plants and accumulate in their tissues. This can potentially lead to the contamination of fruits, vegetables, and grains that we consume, putting our health at risk. Ingesting contaminated food can lead to a variety of health problems, including respiratory issues, neurological disorders, and even cancer.In addition, soil pollution can also impact the quality of our water supply. When contaminated soil comes into contact with groundwater, it can leach harmful chemicals into underground aquifers. This can result in the contamination of drinking water sources, which can have serious consequences for public health.Consuming contaminated water can lead to a variety of health problems, such as gastrointestinal issues, kidney damage, and reproductive disorders.Furthermore, soil pollution can have a negative impact on biodiversity. Contaminated soil can harm plant and animal species that depend on healthy soil for their survival. This can lead to a decrease in biodiversity, disrupting ecosystems and potentially leading to the extinction of certain species. This loss of biodiversity can have far-reaching consequences for our planet, as it can disrupt the delicate balance of ecosystems and harm the overall health of our environment.In conclusion, soil pollution is a serious issue that can have harmful consequences for human health and the environment. It is important for us to take action to prevent soil pollution and protect our soil resources for future generations. By implementing sustainable practices and reducing our reliance on harmful chemicals, we can help mitigate the impact of soil pollution and create a healthier planet for all.篇2Soil pollution refers to the contamination of soil with harmful substances, such as chemicals, heavy metals, andpollutants. This issue is becoming a growing concern for human health and the environment. There are several consequences that soil pollution can bring to human beings.First of all, soil pollution can affect human health. Contaminated soil can lead to the contamination of crops, fruits, and vegetables that grow in it. When people consume these contaminated foods, they may be exposed to harmful substances that can cause various health problems, such as cancer, respiratory diseases, and neurological disorders. In addition, contaminated soil can also contaminate water sources, further increasing the risk of health problems.Secondly, soil pollution can have adverse effects on the environment. Contaminated soil can harm the ecosystem by killing beneficial microorganisms, insects, and plants. This can disrupt the balance of the ecosystem and lead to the extinction of certain species. In addition, soil pollution can also affect the quality of air and water, as pollutants can be released into the atmosphere or leach into groundwater.Furthermore, soil pollution can have economic consequences. Contaminated soil can reduce the productivity of land for agriculture and other purposes. This can lead to a decrease in crop yield, lower quality of produce, and increasedcosts for soil remediation. In addition, soil pollution can also impact property values and lead to financial losses for property owners.In conclusion, soil pollution can have serious consequences for human health, the environment, and the economy. It is important for us to take action to prevent soil pollution and mitigate its effects. This includes implementing proper waste management practices, reducing the use of harmful chemicals, and promoting sustainable agriculture. By working together to address soil pollution, we can protect our health and the environment for future generations.篇3Soil pollution refers to the contamination of soil with harmful substances, such as chemicals, heavy metals, and pesticides. This problem has serious consequences for human health and the environment.First and foremost, soil pollution can have a direct impact on human health. When people come into contact with contaminated soil, either through direct skin contact or by ingesting contaminated food grown in polluted soil, they can suffer from a range of health problems, including skin rashes,respiratory issues, and even cancer. Children are particularly vulnerable to the effects of soil pollution, as they are more likely to play in contaminated soil and put their hands in their mouths.In addition to the health risks posed by soil pollution, it can also have serious environmental consequences. Contaminated soil can affect the fertility of the land, making it difficult to grow crops and sustain plant and animal life. This can lead to a decrease in biodiversity and disrupt ecosystems.Furthermore, soil pollution can also impact water quality. When contaminated soil comes into contact with water sources, such as rivers, lakes, and groundwater, it can leach harmful substances into the water, contaminating it and making it unsafe for human consumption. This can lead to widespread water pollution and pose a serious threat to public health.In conclusion, soil pollution is a serious problem that has far-reaching consequences for both human health and the environment. It is important for governments, industries, and individuals to take action to prevent soil pollution and protect our soil for future generations. By implementing strict regulations, promoting sustainable farming practices, and cleaning up contaminated sites, we can mitigate the effects of soil pollution and create a healthier environment for all.。
蚯蚓在土壤污染修复中的应用
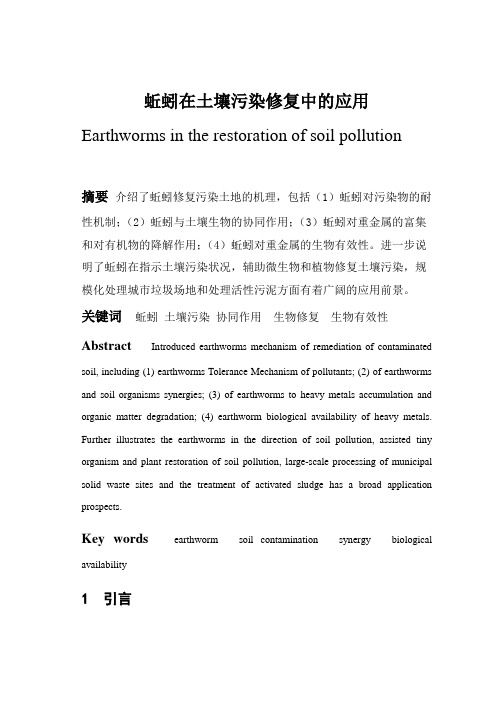
蚯蚓在土壤污染修复中的应用Earthworms in the restoration of soil pollution摘要介绍了蚯蚓修复污染土地的机理,包括(1)蚯蚓对污染物的耐性机制;(2)蚯蚓与土壤生物的协同作用;(3)蚯蚓对重金属的富集和对有机物的降解作用;(4)蚯蚓对重金属的生物有效性。
进一步说明了蚯蚓在指示土壤污染状况,辅助微生物和植物修复土壤污染,规模化处理城市垃圾场地和处理活性污泥方面有着广阔的应用前景。
关键词蚯蚓土壤污染协同作用生物修复生物有效性Abstract Introduced earthworms mechanism of remediation of contaminated soil, including (1) earthworms Tolerance Mechanism of pollutants; (2) of earthworms and soil organisms synergies; (3) of earthworms to heavy metals accumulation and organic matter degradation; (4) earthworm biological availability of heavy metals. Further illustrates the earthworms in the direction of soil pollution, assisted tiny organism and plant restoration of soil pollution, large-scale processing of municipal solid waste sites and the treatment of activated sludge has a broad application prospects.Key words earthworm soil contamination synergy biological availability1引言土壤是人类生态环境的重要组成部分, 是人类社会的生存基础[1],伴随着社会经济的快速发展,人类通过工农业的生产活动,日常生活向土壤排放大量的污染物,超过了土壤的自净能力,使土壤不堪重负,给土壤带来了巨大的灾难。
- 1、下载文档前请自行甄别文档内容的完整性,平台不提供额外的编辑、内容补充、找答案等附加服务。
- 2、"仅部分预览"的文档,不可在线预览部分如存在完整性等问题,可反馈申请退款(可完整预览的文档不适用该条件!)。
- 3、如文档侵犯您的权益,请联系客服反馈,我们会尽快为您处理(人工客服工作时间:9:00-18:30)。
Remediation of soil contaminated with lubricating oil by extractionusing subcritical waterMohammad Nazrul Islam a,Young-Tae Jo a,Jeong-Hun Park a,b,*a Department of Environmental Engineering,Chonnam National University,Gwangju500-757,Republic of Koreab Soil Technology Research Institute,Chonnam National University,Gwangju500-757,Republic of Korea1.IntroductionSubcritical water extraction(SCWE)has become a greenextraction method for the remediation of different classes ofcompounds contaminated soil and is a technique based on theuse of superheated water(1008C T3748C and pressur-e<22.1MPa)as a solvent instead of organic chemicals.Theunique characteristics of subcritical water have been described inthe literature[1–3].Lagadec et al.[4]were among thefirst to fullyexploit the feasibility of SCWE technology to remediate the organiccontaminated sites.Their pilot scale study of hot water extractionof polycyclic aromatic hydrocarbons(PAHs)and pesticides fromsoils was unique in one respect;hot water extraction wascompared to bioremediation and supercritical CO2extraction.Removal rates during one year of bioremediation were much lowereven for low molecular weight PAHs than those obtained after60min of hot water extraction.The PAHs and pesticide contami-nated soils used in their study could not support plant growth priorto treatment with hot water extraction,but both soils were fertilewithout additional treatment after extraction.In addition,a fewresearch groups have reported that SCWE technology can be usedto treat organic contaminants such as PAHs[3,5],polychlorinatedbiphenyls(PCBs)[6,7],pesticides[8],and explosives[9]and haveshown the feasibility of extracting contaminants from contami-nated soils.These water based solvents have significant potentialfor beneficial use in various pollutant removal applications.Contamination of soils by petroleum hydrocarbons(PHCs)poses a major environmental problem,especially to the soilenvironment,and has caused critical health defects;increasingattention has therefore been paid for developing innovativetechnology to clean up this contamination.A recent work by Tanget al.[10]evaluated the eco-toxicity of PHCs contaminated soil andreported that the contaminated soil greatly inhibited seedgermination when the concentration of petroleum was higherthan0.1%,and that0.5%should be considered the critical value forliving microorganisms.Similar concluding remarks have beenreported by other researchers[11,12].Soil polluted with PHCs is often observed as a result of leakingfuel storage tanks,crude oil spills,and the disposal of refinerywaste and railroad cars.Railroad soil or the soil near railroadjunctions usually becomes contaminated with lubricant becausethe railroad industry uses diesel oil for fuel,lubricating oil formachinery,and waste-lubricating oil on the railroad.Such sitesJournal of Industrial and Engineering Chemistry xxx(2013)xxx–xxxA R T I C L E I N F OArticle history:Received5March2013Received in revised form26June2013Accepted27July2013Available online xxxKeywords:Soil remediationLubricating oilSubcritical waterTemperatureRemoval efficiencyA B S T R A C TThe remediation of lubricating oil contaminated soil was investigated by extraction using subcriticalwater.The effects of temperature and time on extraction efficiency were studied by performing eightindividual extractions and varying the subcritical water temperature(200,225,250,and2758C)andextraction time(90,120,180,and240min)in a dynamic mode.Also,a comparison was carried out of thefeasibility of two operational modes,namely,dynamic and static-dynamic mode.Of the25,088mg/kg oflubricating oil as the total petroleum hydrocarbon(TPH)concentration in untreated soil,the residualconcentration was found to be$500and235mg/kg for after120min extraction in a lab-scale apparatusand150min extraction in a30-fold scale-up experiment,respectively,at2758C in static-dynamic mode.The result of this study showed the significant effect of the static-dynamic mode on extraction efficiency.The time and volume of water needed for the static-dynamic mode were much lower than those neededfor the dynamic mode.These results are of practical interest in developing the subcritical waterextraction technology for extraction of lubricating oil and,in a broad sense,petroleum hydrocarbonscontaminated soil.ß2013The Korean Society of Industrial and Engineering Chemistry.Published by Elsevier B.V.All rightsreserved.*Corresponding author at:Department of Environmental Engineering,ChonnamNational University,Gwangju500-757,Republic of Korea.Tel.:+82625301855;fax:+82625301859.E-mail addresses:mnazrul09@(M.N.Islam),tlmanager@(Y.-T.Jo),parkjeo1@jnu.ac.kr(J.-H.Park).Contents lists available at ScienceDirectJournal of Industrial and Engineering Chemistryj ou r n al h o m e p a g e:w w w.e l se v i e r.co m/l oc a t e/j i e c1226-086X/$–see front matterß2013The Korean Society of Industrial and Engineering Chemistry.Published by Elsevier B.V.All rights reserved./10.1016/j.jiec.2013.07.040often contain organic contaminants including benzene,toluene,ethylbenzene,and PAHs originating from PHCs [13];they thus require remediation via a clean-up to protect human health and the ecosystem.Numerous researches have been conducted on the remediation of PHCs contaminated soil using a bioremediation process [14–18].However,bioremediation is not suitable in treating lubricating oil contaminated sites because the oil is highly viscous and does not biodegrade easily [13].In addition,the total petroleum hydrocar-bon (TPH)removal is frequently poor because biodegradation in soil can be limited by many factors,such as microorganism types,nutrients,pH,temperature,moisture,oxygen,soil properties,and contaminant presence [19].While supercritical ethane,supercriti-cal argon,organic solvent,and surfactant remediation processes have proven to be an excellent technique for both extraction and chromatography [20–24],the supercritical water extraction process requires a high temperature of >3748C and pressure of !22.1MPa and is corrosive,while other processes require large volumes of organic solvents that are not only flammable but often toxic or carcinogenic,expensive,and environmentally unfriendly.Electrokinetic (EK)soil remediation has attracted interest among researchers over the last decade.Since 1996,when the first EK remediation was introduced in Korea for soil contaminated with heavy metals,many researchers have investigated different EK system configurations to achieve enhanced performance [25].In recent,modified EK system,namely EK-Fenton remediation has been reported as a promising technique to remediate diesel contaminated soil [26,27].Pazos et al.[26]reported that the TPH removal efficiency of 90%was obtainable by surfactant EK-Fenton process after 15days of treatment.However,this technique is not suitable for remediation of high range hydrocarbons (heavy oil)as well as requires a long treatment time.For example,Park et al.[13]documented the feasibility of EK technology on the remediation of railroad soil contaminated by lubricating oil and zinc;and the removal efficiency of lubricating oil was only $50%after 17days of operation.As a result,a suitable remediation method is needed to treat PHCs contaminated soils.A study by Kim and Kweon [28]showed that oily lubricating materials were effectively removed from the parts of a lubricating machine by SCWE at a relatively low operating temperature.But,so far,the remediation of used lubricating oil contaminated soil by extraction using subcritical water has not been studied.Therefore,the main objective of this research was to exploit the possibility of the SCWE of lubricating oil as an excellent remediation technique to treat soils contami-nated with PHCs.A secondary aim of this study was to optimize the extracting process using subcritical water for the pilot scale application.2.Experimental2.1.Soil characterizationThe main properties assessed in the soil were the pH,the organic matter content and the particle size distribution.The non-polluted soil was collected from Hwasun in South Korea and a soil sample was air-dried,homogenized,and sieved using a 10-mesh sieve.The physicochemical properties of the soil are summarized in Table 1.2.2.Soil contamination procedureA <2mm fraction of 5kg of non-polluted soil sample was contaminated by mixing the soil with used lubricating oil (density =0.8364g/cm 3)that was collected from a car repairing centre nearby our university campus.Lubricating oil was selectedsince it is known as the most difficult contaminant to extract from soil due to its high boiling point fractions (>3508C).Used lubricating oil was previously dissolved in dichloromethane before it was spiked into the soil.135g of used lubricating oil,dissolved in 3L dichloromethane,was added to 5kg soil sample.The soil–dichloromethane mixture was shaken overnight and subsequently the dichloromethane was evaporated by air-drying the soil for about 6h.The contaminated soil was kept in a closed aluminium container at room temperature for 3week to allow the dispersion and sorption of the contaminant in the soil matrix.The average initial concentration of lubricating oil after contamination was 25,088mg/kg,in terms of TPH.It should be noted that the hydrocarbon components of lubricating oil are classified within the chromotographable hydrocarbon range of C 24–C 36.2.3.SCWE equipment and extraction processOne semi-pilot and one lab-scale SCWE apparatus were used in this work to carry out the extractions of the lubricant contaminat-ed soil,in which a lab-scale apparatus previously used for PAHs [5]is described in Fig.1.In the case of the lab-scale apparatus,the stainless steel tube (1mm o.d.,0.6mm i.d.),which is part of the equipment,was used as a heating coil and a water flow line through the pre-heater,main-heater,and condenser to the outer chamber.A high pressure pump (Series II,Chrom Tech,Inc.)was used to deliver water to the pre-heater,followed by a 9.5mL extraction cell (17.0mm o.d.,10.0mm i.d.,and 108.7mm long;made of stainless steel)and the cell was capped at the inlet and outlet with a 2m m stainless steel microfilter.In the case of scale-up extraction,254mL of the extraction cell was constructed from stainless steel (30.0mm o.d.,70.9mm i.d.,and 359.0mm long)with end cap (20m m stainless steel microfilter).An Annovi Reverberi pump (RC-M.01.10,made in PR China)was used in the dynamic flow mode to pump water through the preheating coil of 2.0mm i.d.(4.1mm o.d.)stainless steel tubing and extraction cell.For both extractions,the soil sample was weighed and inserted into the extraction cell which was placed into the main heating chamber.The extraction cell was then closed tightly and connected to the water and contaminant mass flow tube.Thereafter,the water was pumped at a desired flow rate for a desired period through the pre-heating coil and extraction cell.The temperature in the preheating coil and extraction cell was observed by a thermocouple connected to a West 6100+temperature controller (Ise,Inc.,USA).Superheated water has a decreased dielectric constant,surface tension,and viscosity,thus making it an efficient solvent for extracting soluble poor or non-soluble organic contaminants while passing through the extraction cell.A system pressure regulator valve was used to control and allow the pressure to build up.The extraction cell was heated to the desired temperature.After the desired extraction,the pump and heater were stopped and the pressure was released to atmospheric pressure.The reactor was left to cool to roomTable 1Physicochemical properties of used silt loam soil.PropertiesValuespH6.62Total organic matter (%)9.23Particle distribution (%)Sand 38Silt 59Clay3Initial concentration aLubricating oil (mg/kg)as TPH25,088aInitial concentration was based on the analysis of three 10-g subsamples of a homogenized soil sample.M.N.Islam et al./Journal of Industrial and Engineering Chemistry xxx (2013)xxx–xxx2temperature and opened to collect the soil to determine the residual concentrations of lubricating oil.One duplicate extraction procedure was performed for each of the experimental condition conducted in this study.2.3.1.Dynamic SCWE procedureA 12g soil sample was weighed and placed into the extraction cell and then into the extraction chamber in all experiments.Note that the lab-scale apparatus was used for this procedure.After assembling the unit of extraction system,the unit was filled with water pumped at a flow rate of 1mL/min by closing the outlet valve (in this way the system was pressurized to 6MPa)and turned on the heating valve.When the temperature inside the cell was 1008C,the outlet valve was then opened and 0.3mL/min of flow-rate was enabled until the inside temperature of the cell raised to the desired level (e.g.2758C).After the cell inside temperature reached the set temperature,the counting of extraction time began and dynamic extraction was then performed by pumping the water at 1mL/min during the desired extraction time.2.3.2.Static-dynamic SCWE procedureBoth a lab-scale and semi-pilot apparatus were used for static-dynamic mode extraction.This extraction mode involved the following steps:(1)the same sample amount (12g)as the dynamic mode extraction (360g for 30-fold scale-up extraction)was placed in the extraction cell;(2)the unit was assembled and filled with water by closing the outlet valve (in this way the system was pressurized to 6MPa)and temperature switched on;(3)when the cell inside temperature was reached 1008C,the outlet valve was then opened and 0.3mL/min of water flow (9mL/min for 30-fold extraction)was allowed until the inside temperature of the cell raised to the desired value;(4)after the inside temperature of the cell reached 2758C and the system pressure reached 6MPa,the static extraction step was developed for 15min by closing the inlet and outlet valves;(5)after this time had elapsed,both the inlet and outlet valves were opened,and the high pressure pump was switched on,driving the water through the system at a 1mL/min (30mL/min in the case of 30-fold scale-up extraction to maintainthe same residence time of water within the extraction cell)flow-rate for 15min (dynamic extraction step);(6)the static and dynamic steps were developed consecutively for the desired extraction period.2.4.Analytical procedureThe method of determining the amount of lubricating oil in the corresponding soil samples was based on the Korean Standard Test Method:10g of soil was mixed with anhydrous sodium sulfate and was then ultrasonically extracted twice with 200mL of dichloromethane in 100mL dichloromethane for 3min each time.The extract was filtered and extractant was concentrated using a rotary evaporator and purified with silica gel.The amount of TPH in the final solution was analyzed using gas chromatography fitted with a flame ionization detector (HP-6890,Agilent Tech.,USA).3.Results and discussionRemediation of soil contaminated with PHCs at an industrial scale remains a challenge.Thus,as a preliminary step in evaluating subcritical water as a useful extraction solvent for PHCs and to optimize the extraction process,spiking experiments were conducted with silt loam soil at a laboratory scale.The effects of flow rate and pressure in SCWE were not studied in detail.In many studies,the flow rate of 1mL/min for lab-scale extraction (using 8–12g of contaminated soil)has been found to be appropriate [4,29].The purpose of maintaining particularly high pressure in SCWE was to maintain water in a liquid state when the temperature was increased.Chang et al.[30]found that pressure had only a minor effect on the removal efficiency at 2008C and similar findings were reported by Hawthorne et al.[7].However,previous works from the literature and results from our laboratory concur about the best conditions of temperature and extraction time to reach high extraction efficiencies of organic pollutants [5,6,31].The effects of temperature and time on the removal of lubricating oil were therefore investigated using 1mL/min of water flow rate at a constant pressure of 6MPa.Fig.1.Subcritical water extraction apparatus.PR:pressure relief valve;PI:pressure indicator;T:thermocouple.M.N.Islam et al./Journal of Industrial and Engineering Chemistry xxx (2013)xxx–xxx33.1.Effect of temperature and time on lubricating oil removalThe operating conditions to be optimized were selected as extraction temperature in the range of 200–2758C and four levels of time (90,120,180and 240min)in dynamic mode.Although it is believed that subcritical water above a temperature of !3008C may facilitate the extraction efficiency of lubricating oil,this makes it difficult to use at a large scale with high pressure.Fig.2a shows the lubricating oil removal from the soil according to the water temperature,in which each particular extraction experiment was conducted for 90min.A positive relationship was shown between temperature and lubricating oil extractability (R 2=0.97).It is clearly observed that the reduction of TPH concentration was $3.5-fold when the increase in temperature was from 200to 2758C (the concentration reduction in percent is equivalent to the extraction or removal efficiency).Temperature is an important parameter in SCWE since the decreased dielectric constant and surface tension of subcritical water allows for significant dissolution of nonpolar organic compounds in water at elevated temperature [2],thus,solubility of PHCs is greatly enhanced with increasing temperature.When water at a temperature of 258C (the water did not maintain any additional pressure)passed through the extraction cell,<1%of the lubricating oil was removed from the soil (data not shown)after 90min for a flow rate of 1mL/min.The removal efficiency of lubricating oil at a maximum of 2758C was determined since the water heating unit in the scale-up system was capable of heating to 2758C.After 90min extraction using a flow rate of 1mL/min,the TPH concentration of same polluted soil reduced from 25,088to 12,042mg/kg,which is only 52%of removal efficiency observed.The residual concentration value is so far to meets the current environmental legislation value of the Korean government (2000mg/kg).An optimum temperature of 2758C was selected for subsequent extraction with an increase in the extraction time.It is expected that a longer extraction time increases the removal efficiency of lubricating oil in soil.According to Richter et al.[32],the dynamic time on the extraction efficiency was somewhat important in terms of kinetics and soil/water equilibri-um.As shown in Fig.2a,the maximum removal of lubricating oil was observed at 2758C since it was not at a satisfactory level even after 90min extraction.Therefore,additional experiments were performed with the three levels of extraction time of 120,180,and 240min (the counting of extraction time started after the reactor temperature reached the set temperature of 2758C)and other parameters were kept constant (water flow rate 1mL/min and pressure 6MPa).The results showed that there was an increasing trend in the yield of lubricating oil extraction with the increase of time in the range of 90–180min (Fig.2b),but there was no significant increase while the extraction time was extended to240min,revealing the high persistence of lubricant in soil.Also,this observation suggests that a considerable amount of time is required for the release of lubricant by the SCWE system.When the extraction temperature was 2758C and the flow rate was 1mL/min,the removal of lubricating oil as a TPH was 62%after 180min.The present results show that a significant part (62%)of the lubricating oil is removed by the SCWE mechanism after 180min extraction at 2758C.Clearly,this amount is not sufficient to fully ‘remediate’heavily contaminated soil.Also,it is believed that the lubricating oil from aged soils would be more difficult,due to the stronger interaction with the soil matrix.3.2.Kinetic study of lubricating oil removalIn order to determine the extraction pattern of lubricating oil and a more quantitative account of removal efficiency with increasing time,a kinetic study was carried out in which the experimental data were fitted with the first-order kinetic desorption model.The applied model was described briefly in our previous study for SCWE of PAHs [33]and elsewhere [23,34].The plot of the measured TPH removal concentration according to time using the kinetic model illustrated the lubricating oil desorption from soil.The model used in this study was expressed by the following equation:C tC 0¼1Àe ðÀk ;t Þwhere C t (mg/kg)is the amount of lubricating oil removed at instant t ,C 0(mg/kg)is the concentration of TPH in spiked soil (untreated),k (min À1)is the desorption rate constant,and t (min)is the time of extraction.Microsoft excel solver was used to fit the experimental data with kinetic model to obtain the rate constant k value.The value of k is presented in Table 2.The first order model fitted the experimental results based on the square regression coefficient,R 2;the SCWE of lubricating oil in the case of soil remediation can thus be described by a first-order kinetic model.As shown in Fig.3,the predicted value of TPH removal of over 90%could be achieved after 9h extraction.Indeed,although the extraction conditions became harsher with an increasingdynamicFig.2.(a)Effect of temperature on the extraction of lubricating oil from contaminated soil in which the constant extraction time was maintained for 90min and (b)the effect of time on extraction in which the constant subcritical water temperature was 2758C.All experiments were conducted in dynamic mode using 12g of soil at a constant 1mL/min flow of water and 6MPa of pressure.Table 2First-order desorption kinetic coefficient.Parameter Value k (min À1)0.0045R 20.96M.N.Islam et al./Journal of Industrial and Engineering Chemistry xxx (2013)xxx–xxx4time of up to 9h,there was an increase in the amount of compound extracted in terms of the requirement for a large volume of water and energy.Note that,according to this predicted removal requires a large volume of subcritical water,after 9h extraction,$570mL of water is needed for a 12g sample.3.3.Effect of static-dynamic mode on TPH removalTwo operational modes were used to carry out extraction with subcritical water:the dynamic mode and the static-dynamic mode.In the dynamic mode,the subcritical water flows continually through the extraction cell.In the static-dynamic mode,a fixed volume of water was kept within the extraction cell for 15min by closing the outlet valve (static mode);after this time had elapsed,the outlet valve was opened and a high pressure pump was switched on,driving the subcritical water through the system for 15min (dynamic mode)under a working temperature of 2758C and pressure of 6MPa.The extraction mechanism of contaminants with static-dynamic cycling in SCWE could be described by a combination of equilibrium and separation steps.During the static soak time,the system was allowed ample time to reach equilibrium,and was then released during the dynamic period as fresh solvent (water)was periodically pumped through the extraction cell.In the static-dynamic mode extraction,a single static extraction for 15min followed by a 15min dynamic extraction were combined as one cycle and there were four cycles in each run,resulting in a total extraction time for the static-dynamic mode of 120min.Table 3shows the residual concentration of TPH at different extraction conditions for the dynamic mode andstatic-dynamic mode.Examining the TPH in soil after 120min of static-dynamic extraction that met the regulation level of the Korean government;however,the residual concentration was much higher after the same period of dynamic mode extraction.The results in Table 3shows a significant positive impact of the static-dynamic mode on the extraction of lubricating oil from soil,in which the removal was approximately twice that of dynamic extraction under the same condition,indicating that the extraction efficiency depends mostly on contact time rather than water volume.This result concurs with that from various other previous studies as outlined below.Comparative studies have been conducted of the static,dynamic,and static-dynamic modes for the analytical purpose of determining PAHs in solid samples with pressurized hot water extraction [35,36].Luque-Garcia and Luque de Castro [35]analyzed the nitrated PAHs in both spiked and natural contaminated soils,showing that the static-dynamic mode is the most suitable alternative in terms of quantity extracted (based on quantitative analysis)of PAHs and total extraction time shorten.They reported that the static-dynamic mode only required 25min for total extraction of the analytes,while the static and the dynamic mode required 30min and 50min,respectively.According to Ghoreishi and Shahrestani [37],the residence time (the average time needed for the water to remain in an extraction cell)could be a function of extraction yield in a subcritical water extraction system and a lower extraction can be predicted to decrease the residence time due to continuous and/or higher flow rates.Aksu et al.[38]observed that for biosorption of reactive dyes on Rhizopus arrhizus in a continuous fixed bed reactor,if the residence time of the solute in the column is too short,the total amount of sorbed dye is decreased.In this study,the increase in removal efficiency at static-dynamic mode with ample residence time indicates that the removal efficiency is controlled by intraparticle diffusion and/or mass transfer.The removal efficiency was over 98%after 120min static-dynamic extraction,indicating that the intraparticle diffusion and mass transfer is well balanced with external mass transfer (advection)rate when employing a periodical flow condition.In our study,a significant difference was observed between the dynamic and static-dynamic extraction on TPH removal.In this regard,one possible explanation for the effect of dynamic water flow is the formation of a preferential pathway (channelling)in the cell,and parts of the lubricant in the soil do not come in contact with advection flow.In other words,different mass transfer zones (advection or diffusion dominant zone)can be formed.Desorbed contaminants can easily be removed in an advection dominant zone where channelling flow occurs.However,desorbed contaminants in a diffusion dominant zone cannot be easily removed,as shown by the low removal efficiency in the dynamic mode.On the other hand,in the static-dynamic extraction,during the static period,the pressurized stagnant liquid penetrated and dispersed through the entire area,causing diffusion and advection transport from all parts of the soil,resulting in increasing the mass transfer from the internal to the external bulk fluid.This annotation is consistent with similar observations by Pavlostathis and Jaglal [39]who reported that the slow desorption and/or diffusion rates compared to the advective rate under continuous pumping may account for the slow release of trichloroethane (TCE)from a contaminated soil in a column reactor.In this study,a 50%reduction in the volume of effluent (extract)was obtained by static-dynamic extraction compared to dynamic mode extraction.The total removal of lubricating oil was achieved in less time than that required by the dynamic mode,thus a small volume of wastewater wasproduced.Fig.3.Experimental fitting of first-order kinetic desorption model and predicted removal percentage of lubricating oil.Table 3Results of SCWE at different conditions.Run aExtraction modeExtractiontime (min)/no.of cycleAverage residual conc.in soil (mg/kg)Removal efficiency (%)Lab-scale extraction (12g of soil)1Dynamic 120/–12,042522Static-dynamic 120/4503b 9830-Fold extraction (360g of soil)3Static-dynamic 120/47661694Static-dynamic 150/5235b99aAll experiments were conducted at 2758C (6MPa).Duplicate extraction was carried out for each of the experimental condition with an experimental error less than 5%.bThe residual concentration was much below the pollution limit (2000mg/kg).M.N.Islam et al./Journal of Industrial and Engineering Chemistry xxx (2013)xxx–xxx5。