Root responses to cadmium in the rhizosphere
采用流式细胞仪鉴定紫花苜蓿花药愈伤组织的变异

第 2 0卷 第 3 期
草 业 学 报 2 1 年 01
1 2 苜蓿花 药愈 伤组 织 的诱 导培 养 . 在苜 蓿初 花期 到盛 花期 期 间 , 集 3个基 因型 紫花 苜蓿 单 株上 花 药 发育 处 于单 核 中、 采 晚期 的花 蕾 ( 用 醋酸 使 洋红 染 色压 片法 , 观察 、 定发 育 时期 。从 外 观上 选择 花冠 最外 面 的旗瓣 紧包 , 冠 饱满 , 出花萼 1 确 花 伸 ~2mm, 呈 刀尖 状 的花蕾 ) 。将采 集 的花蕾 放 置在 4 的冰 箱 内低温 处 理 1 ~2 ; ℃ 2 4h 然后 将 花 蕾 灭菌 , 无 菌 条件 下 剥 出花 在 粉, 接种 到愈 伤组 织诱 导 培养基 中( +0 5g L 2 4D+0 2g L NAA+0 5g L 6B NB . / ,一 . / . / - A+ 2g L K / T+9 蔗糖 +0 7 琼脂 ) 在 温度 为 2 。 光 强 10 0l 光 照 时间 1 / 、 . , 5C、 0 x、 6h d 湿度 4 的环 境下 培 养 。诱 导 出愈 伤组 织 后转 0 入继 代 培养基 ( NB+3/ 糖 +0 7 琼脂 ) 2 6 9蔗 . ,0d为 1个继代 周 期 。培养 条件 同上 。
色 3 ~ 6 , 成 细胞核 染 色 , 胞核 悬浮 液制 备完 成 。 O OS完 细 2 以已知 的 四倍 体 苜蓿 叶片 ( n z 2 为对 照 。调 整 分 析仪 的放 大 倍 数 , 对 照 的 D ) 2 一4 一3 ) 使 NA 峰 值 处 在 2 0 0 道 附 近 。峰值 处在 1 0道 的样 本 为二倍 体 , 0 而处 在 3 0和 4 0道 的分别 为三 倍体 和八倍 体 , 0 0 其余 的 为嵌合 体 。嵌 合 体 又根据 峰值 位 置确 定其 构成 细胞 的倍 性 。 3 将 1 所得 细胞 核悬 浮液 用调 整好 的流 式 细胞仪 进行 检测 , NA 含量 的分 布 曲线 图 由仪 器 自动生 成 。 ) ) D
氯化锂对地塞米松诱导的大鼠胰岛β细胞凋亡的影响及其机制
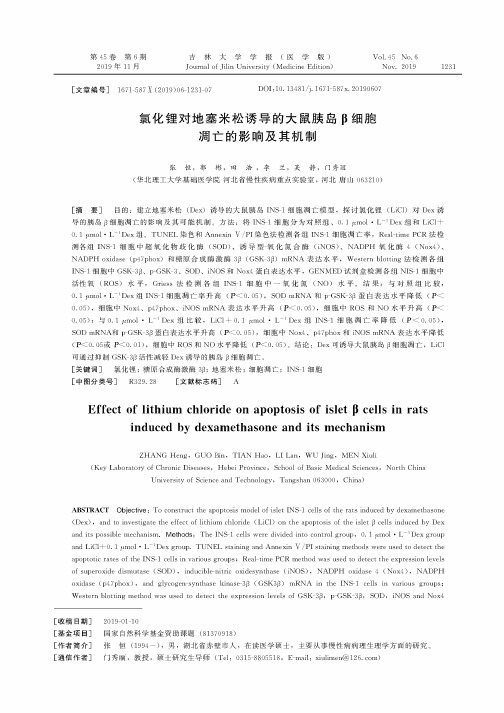
ABSTRACT Objective: To construct the apoptosis model of islet INS-1 cells of the rats induced by dexamethasone (Dex) , and to investigate the effect of lithium chloride (LiCl) on the apoptosis of the islet 4 cclls induced by Dex and its possible mechanism. Methods: The INS-1 cells were divided into control group, 0. 1 gmol • L_lDex group and LiCl+0. 1 gmol • L_l Dex group. TUNEL staining and Annexin V /PI staining methods were used to detect the apoptotic rates of the INS-1 cells in various groups ; Real-time PCR method was used to detect the expression levels of superoxide dismutase (SOD), inducible-nitric oxidesynthase (iNOS) , NADPH oxidase 4 (Nox4), NADPH oxidase (p47phox), and glycogen-synthase tinase-30 (GSK30) mRNA in theINS-1 cclls in various groups; Western blotting method was used to detect the expression levels of GSK-34, p-GSK-30, SOD, iNOS and Nox4
土壤英文文献
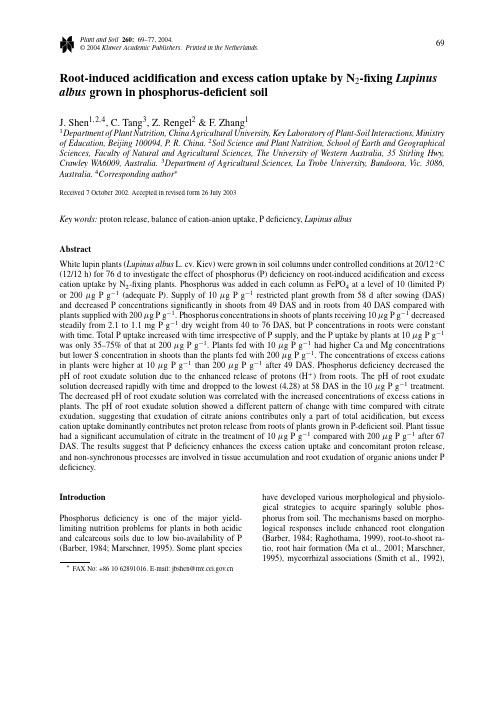
Plant and Soil 260: 69–77, 2004. © 2004 Kluwer Academic Publishers. Printed in the Netherlands.69Root-induced acidification and excess cation uptake by N2 -fixing Lupinus albus grown in phosphorus-deficient soilJ. Shen1,2,4 , C. Tang3 , Z. Rengel2 & F. Zhang11 Department of Plant Nutrition, China Agricultural University,Key Laboratory of Plant-Soil Interactions, Ministry of Education, Beijing 100094, P. R. China. 2 Soil Science and Plant Nutrition, School of Earth and Geographical Sciences, Faculty of Natural and Agricultural Sciences, The University of Western Australia, 35 Stirling Hwy, Crawley WA6009, Australia. 3 Department of Agricultural Sciences, La Trobe University, Bundoora, Vic. 3086, Australia. 4 Corresponding author∗Received 7 October 2002. Accepted in revised form 26 July 2003Key words: proton release, balance of cation-anion uptake, P deficiency, Lupinus albusAbstract White lupin plants (Lupinus albus L. cv. Kiev) were grown in soil columns under controlled conditions at 20/12 ◦ C (12/12 h) for 76 d to investigate the effect of phosphorus (P) deficiency on root-induced acidification and excess cation uptake by N2 -fixing plants. Phosphorus was added in each column as FePO4 at a level of 10 (limited P) or 200 µg P g−1 (adequate P). Supply of 10 µg P g−1 restricted plant growth from 58 d after sowing (DAS) and decreased P concentrations significantly in shoots from 49 DAS and in roots from 40 DAS compared with plants supplied with 200 µg P g−1 . Phosphorus concentrations in shoots of plants receiving 10 µg P g−1 decreased steadily from 2.1 to 1.1 mg P g−1 dry weight from 40 to 76 DAS, but P concentrations in roots were constant with time. Total P uptake increased with time irrespective of P supply, and the P uptake by plants at 10 µg P g−1 was only 35–75% of that at 200 µg P g−1 . Plants fed with 10 µg P g−1 had higher Ca and Mg concentrations but lower S concentration in shoots than the plants fed with 200 µg P g−1 . The concentrations of excess cations in plants were higher at 10 µg P g−1 than 200 µg P g−1 after 49 DAS. Phosphorus deficiency decreased the pH of root exudate solution due to the enhanced release of protons (H+ ) from roots. The pH of root exudate solution decreased rapidly with time and dropped to the lowest (4.28) at 58 DAS in the 10 µg P g−1 treatment. The decreased pH of root exudate solution was correlated with the increased concentrations of excess cations in plants. The pH of root exudate solution showed a different pattern of change with time compared with citrate exudation, suggesting that exudation of citrate anions contributes only a part of total acidification, but excess cation uptake dominantly contributes net proton release from roots of plants grown in P-deficient soil. Plant tissue had a significant accumulation of citrate in the treatment of 10 µg P g−1 compared with 200 µg P g−1 after 67 DAS. The results suggest that P deficiency enhances the excess cation uptake and concomitant proton release, and non-synchronous processes are involved in tissue accumulation and root exudation of organic anions under P deficiency.Introduction Phosphorus deficiency is one of the major yieldlimiting nutrition problems for plants in both acidic and calcareous soils due to low bio-availability of P (Barber, 1984; Marschner, 1995). Some plant species∗ FAX No: +86 10 62891016. E-mail: jbshen@have developed various morphological and physiological strategies to acquire sparingly soluble phosphorus from soil. The mechanisms based on morphological responses include enhanced root elongation (Barber, 1984; Raghothama, 1999), root-to-shoot ratio, root hair formation (Ma et al., 2001; Marschner, 1995), mycorrhizal associations (Smith et al., 1992),70 and decreased root radius (Föhse et al., 1991). In addition, physiological strategies are characterized by enhanced rhizosphere acidification (Hedley et al., 1982; Hinsinger et al., 2003; Marschner, 1995; Tang et al., 2001), and increased root exudation of organic chelates (Dinkelaker et al., 1989; Gardner et al., 1983; Jones, 1998; Ryan and Delhaize, 2001), reducing agents and phosphatase (Dracup et al., 1984; Neumann et al., 1999, 2000). White lupin (Lupinus albus L.) is an important grain and forage crop widely grown in infertile soils. Under P deficiency, white lupin increases the formation of cluster roots, release of H+ and exudation of citrate (Dinkelaker et al., 1989; Gardner et al., 1983; Lamont, 2003; Neumann et al., 1999). For example, the enhanced net release of H+ from cluster roots acidified rhizosphere from pH 7.5 to 4.8 (Dinkelaker et al., 1989, 1995) and even as low as pH 3.6 in some cases (Li et al., 1997). Citrate concentration in root cluster rhizosphere can reach as high as 100 µmol g−1 soil, and the amount of citrate exudation from roots represented up to 23% of the total plant dry weight (Dinkelaker et al., 1989, 1995; Gerke et al., 1994). Large amounts of proton release and citrate exudation may facilitate P acquisition by white lupin from sparingly soluble P in soils (Gerke et al., 1994; Hinsinger, 1998; Marschner, 1995; Raghothama, 1999). Rhizosphere acidification and citrate exudation induced by P deficiency have been studied mostly in white lupin grown in nitrate-containing nutrition solutions (Gardner et al., 1983; Johnson et al., 1994, 1996a, b; Keerthisinghe et al., 1998; Neumann et al., 1999; Neumann and Römheld, 1999; Shane et al., 2003; Watt and Evans, 1999a, b), or in soil culture with nitrogen supply as nitrate (Dinkelaker et al., 1989; Kamh et al., 1999) or ammonium nitrate (Li et al., 1997). Nitrogen forms have a marked influence on rhizosphere acidification due to influencing the balance of anion and cation uptake by plants (Hinsinger, 2001; Hinsinger et al., 2003; Marschner, 1995). Only a few studies used N2 -fixing P-deficient white lupin plants to elucidate the pH changes caused by H+ release and root exudation (Sas et al., 2001; Shen et al., 2003; Tang et al., 1997, 2001). The legume plants dependent on N2 -fixation could take up excess cations over anions, resulting in enhanced H+ release with an increasing amount of N2 fixed (Tang et al., 1997, 2001). Moreover, localized rhizosphere acidification and citrate exudation was also reported previously (Dinkelaker et al., 1989; Keerthisinghe et al., 1998; Neumann et al., 1999, 2000). However, littleTable 1. Basic nutrients applied to soil as solution form prior to planting. The nutrients added were thoroughly mixed with soil and then the soil mixture was carefully packed into plastic soil-column pots (diameter×height = 86×380 mm) for planting. There was 3-kg dry soil per pot Chemicals K2 SO4 CaCl2 .2H2 O MgSO4 .7H2 O MnSO4 .H2 O ZnSO4 .7H2 O CuSO4 .5H2 O H3 BO3 Na2 MoO4 .5H2 O CaCl2 .2H2 O MgSO4 .7H2 O Co2 SO4 .7H2 O Application rates (mg pot−1 ) 400 500 130 20 30 6 2 0.5 500 130 1information was available about patterns of pH change caused by H+ release by the whole root system and excess cation uptake of N2 -fixing Lupinus albus grown in P-deficient soil during various growth stages. The objective of the present study was to investigate the effect of P deficiency on root-induced acidification and excess cation uptake by N2 -fixing white lupin at different growth stages, and assess the relationship between balance of anion-cation uptake and H+ release, and organic acid anion exudation under P deficiency.Materials and methods Plant cultivation Seeds of white lupin (Lupinus albus L. cv. Kiev) were germinated and grown in column pots containing 3 kg of air-dried soil. Virgin brown sand (Uc4.22, Northcote, 1971) was collected from a bushland site 15 km south-east of Lancelin, WA (31.56 S, 115.20 E). The soil fertility was characterized as follows: organic carbon of 8.4 g kg−1, available P (Colwell P) of 5 µg g−1 , NO3 − -N of 2 µg g−1 and NH4 + -N of 1 µg g−1 . The soil conductivity was 0.0022 S m−1 , and the soil pH was 5 (CaCl2 ) or 5.7 (H2 O) (Shen et al., 2003). The soil texture is sand with 96% sand, 2% slit and 2% clay. The contents of oxy-hydroxides are very low with 0.54% Fe2 O3 and 0.11% Al2 O3 (Brennan et al., 1980). Phosphorus was added into the soil as FePO4 at71 a rate of 10 (limited P) or 200 µg P g−1 (adequate P). Nitrogen was supplied only via biological N2 fixation through inoculating the seeds with Bradyrhizobium sp. (Lupinus) WU 425 by adding suspension solution (Sas et al., 2001; Tang et al., 2001). Other basic nutrients were added according to Table 1. The experiment was conducted in a controlled glass-house with temperature of 20/12 ◦ C (12-h day/12-h night) and relative humidity of 75–85%. The soil was watered up to 90% of field capacity by weighing pots with plants once or twice daily. Three replicate pots of each treatment were used for root exudate collection, plant harvest, and nutrient uptake and carboxylate analysis at each of five harvests. The pots were completely randomized and repositioned weekly to minimize any effect of uneven environmental factors. for determination of mineral composition including total K, Na, Ca, Mg, P, S and Cl. Measurements The pH of the root exudate solution A 30-mL aliquot of collected solution was subsampled from the collected solution, and the pH was determined using an Orion 940E pH meter with a combined glass electrode. Organic anions Retention times and absorption spectra of 12 organic acid standards (oxalic, tartaric, formic, malic, malonic, lactic, acetic, maleic, citric, succinic, fumaric and aconitic acids) were used to identify the composition of organic anions in tissue extracts or root exudates. The 10-mL samples of plant extracts or root exudates were filtered through sterile Millex GS Millipore 0.22-µm filters and directly analyzed for organic anions on a reversed phase column (Alltima C18 5 Micron, length 250 mm, i.d. 4.6 mm) using Waters HPLC (Shen et al., 2003). Mineral composition of plant tissues Concentrations of total K, Na, Ca, Mg, P and S were determined in roots and shoots after digesting plant materials in a mixture of concentrated nitric and perchloric acids (Johnson and Ulrich, 1959). Concentrations of total K, Na, Ca, Mg and S were determined using inductively coupled plasma emission spectrometry. Phosphorus was assayed using the vanadomolybdate method (Westerman, 1990). Chloride was analysed colourimetrically in the water extract. The concentrations of excess cations [cmol (+) kg−1 ] in plants were calculated as the sum of charge concentration of K+ , Ca2+ , mg2+ and Na+ minus the sum of H2 PO4 − , SO4 2− and Cl− according to the method described by Tang et al. (1997). Statistics Analysis of variance for comparisons among means was conducted using the SPSS statistical software (SPSS, 1998).Sampling of root exudates and plant materials Root exudates were collected by percolating the soil column containing intact plants with deionized water for 2 h (from 10:00 to 12:00 in the morning) at 40, 49, 58, 67 and 76 DAS according to the method described elsewhere (Shen et al., 2003). An aliquot of 600-mL deionized water was percolated through the soil column three times. The gravimetric analysis showed that 300 mL of solution was retained within the soil matrix, and thus the total volume for calculating carboxylate concentrations was 900 mL. The leachate contained water-soluble root exudates and was referred to as the ‘root exudate’. The pH of the leachate was determined immediately after collecting root exudate solution. Afterwards, Micropur (Sicheres Trinkwasser, Germany) at 0.01 g L−1 and three drops of concentrated H3 PO4 were added to the collected root exudates to inhibit the activity of microorganisms. A sub-sample of 10 mL from the collected solution was kept at −20 ◦ C for later analysis of carboxylates. After each root exudate collection, the plants were harvested, and roots and shoots were separated. Extraction of organic acids from plant materials was done according to the method reported by Neumann et al. (1999). Plant tissue samples were homogenized using ceramic mortar with 5% (v/v) H3 PO4 at the ratio of 1 mL H3 PO4 solution to 0.1 g fresh weight of plants. After centrifugation for 10 min at 10 000 g, the supernatant was diluted 10-fold with HPLC-elution buffer and analyzed by reversed-phase HPLC. Shoot and root weights were recorded after drying in an oven at 70 ◦ C for 3 d. After grinding, the plant materials were used72Figure 1. Fresh weights of shoots and roots (g plant−1 ) of white lupin with 10 (limited P) and 200 (adequate P) µg P g−1 soil at various growth stages. Vertical bars on symbols represent means±SE (n = 3 replicate pots). The separate bar represents LSD (P = 0.05) for any two means according to an analysis of variance for different treatments.Results Plant growth A significant difference was observed in fresh weight between 10 and 200 µg P g−1 at 58 DAS for shoots, which occurred earlier than shoot dry weight changes (Shen et al., 2003), and at 67 DAS for roots (Figure 1). From day 58, plants receiving 10 µg P g−1 showed reduced growth as compared with those at 200 µg P g−1 . Fresh weights of shoots of white lupin at 10 µg P g−1 were 83%, 72% and 53%, respectively, of those at 200 µg P g−1 at 58, 67 and 76 DAS. The plants in two P treatments started to flower at 55 DAS, and the number of flowers in plants fed with 200 µg P g−1 was much greater than that at 10 µg P g−1 at 58 DAS. From day 58, the plants at 200 µg P g−1 also grew taller than those at 10 µg P g−1 (data not shown).Figure 2. P concentrations and contents in plants of white lupin grown at 10 or 200 µg P g−1 soil for 76 d. Vertical bars on symbols represent means±SE (n = 3 replicate pots). The separate bar represents LSD (P = 0.05) for any two means according to an analysis of variance for different treatments.Chemical composition of plant tissues The concentrations of P in shoots of plants fed with 10 µg P g−1 were significantly lower than those at 200 µg P g−1 from 49 DAS, and there were the higher concentrations of P in roots of plants receiving 20073 than 10 µg P g−1 from 40 to 76 DAS (Figure 2). Phosphorus concentrations in shoots of plants receiving 10 µg P g−1 decreased steadily from 2.1 to 1.1 mg P g−1 shoot dry weight from 40 to 76 DAS. In contrast, P concentrations in roots supplied with 10 µg P g−1 had no significant changes with time. At 200 µg P g−1 , there was a trend for decreasing P concentrations in both shoots and roots with time from 40 to 76 DAS. The P concentrations in shoots and roots of plants receiving 200 µg P g−1 were significant lower at 76 than at 40 DAS. The higher P concentrations in shoots were observed at 58 compared with those at 49 and 76 DAS. The total P uptake increased with time irrespective of P supply, but the P uptake by the plants at 10 µg P g−1 was only 35–75% of that at 200 µg P g−1 (Figure 2). Plants fed with 10 µg P g−1 soil had higher Ca concentrations in shoots at 49 and 67 DAS, and had higher Mg concentrations in shoots at 49, 67 and 76 DAS (Figure 3). The concentrations of Ca in roots were higher at 67 DAS in the treatment of 10 than 200 µg P g−1 . Plants supplied with 10 µg P g−1 showed higher K concentrations in shoots at 49 DAS, and in roots at 67 and 76 DAS in comparison to plants receiving 200 µg P g−1 . However, Na concentrations in shoots were lower at 40 DAS in the treatment of 10 than 200 µg P g−1 . There was a lower S concentration at 58 DAS and higher Cl concentration at 67 DAS in the treatment of 10 than 200 µg P g−1 . However, there was no significant difference in the concentrations of Na, Mg, S and Cl in roots between P treatments. Ca and Cl concentrations were higher, and Na, Mg and S concentrations were lower in shoots than in roots.Figure 3. Concentrations of K, Na, Ca, Mg, S and Cl in shoots and roots of white lupin grown at 10 or 200 µg P g−1 soil for 76 d. Vertical bars on symbols represent means ±SE (n = 3 replicate pots). The separate bar represents LSD (P = 0.05) for any two means according to an analysis of variance for different treatments.Root-induced acidification Excess cation uptake Growing plants decreased the pH of soil leachate (root exudates) compared with the control without any plants irrespective of P treatments (Figure 4). The pH of root exudate solution in the treatment of 10 µg P g−1 was significantly lower at 58 and 67 than at 40 or 49 DAS. In contrast, for the treatment of 200 µg P g−1 , the pH of root exudate solution remained constant with time. At 58 and 67 DAS, the pH of collected solutions from the plants fed with 10 µg P g−1 was significantly lower than that from plants receiving 200 µg P g−1 . There was no significant difference in the pH of root exudate solution between the treatments of 10 and 200 µg P g−1 at 40, 49 and 76 DAS. The concentrations of excess cations in plants were significantly higher in the treatments of 10 than 200 µg P g−1 at 49 and 76 DAS in shoots, and only at 76 DAS in roots (Figure 5). The concentrations of excess cations in plants tended to be lower at 10 than 200 µg P g−1 soil at 40 DAS despite no significant difference. However, after 49 DAS, the 10 µg P g−1 treatment surpassed the adequate P treatment in the concentrations of excess cations in plants. The highest concentrations of excess cations for the plants grown at 10 µg P g−1 were observed at 49 DAS for shoots and at 58 DAS for roots, and then the concentrations decreased with time.74Figure 4. The pH in root exudate solution of white lupin grown at 10 or 200 µg P g−1 soil for 76 d. Vertical bars on symbols represent means±SE (n = 3 replicate pots). The separate bar represents LSD (P = 0.05) for any two means according to an analysis of variance for different treatments. There was one replicate for the treatments without plants.The plants grown at 200 µg P g−1 showed a continuous decrease in excess cation concentrations in shoots with time, while in roots an increased concentration from 40 to 58 DAS and then a decrease till 76 DAS were observed. There were higher concentrations of excess cations in the whole plants grown at 10 µg P g−1 compared with the plants receiving 200 µg P g−1 after 49 DAS, and the difference in concentrations of excess cations between P treatments was significant at 49 and 76 DAS. Concentrations of carboxylates in roots and shoots of plants and citrate exudation The concentrations of citrate in shoots or roots of the plants fed with 10 µg P g−1 significantly increased with time from 40 to 76 DAS, but remained unchanged for the plants fed with 200 µg P g−1 over the experimental period (Figure 6). The citrate concentrations in shoots and roots were significantly higher in the plants receiving 10 than 200 µg P g−1 after 67 DAS. The increased exudation of citrate from whole root system of white lupin in the limited P treatment was observed compared with the adequate P treatment over the period monitored (Figure 6, calculated from Shen et al., 2003). There was a higher exudation of citrate at 49 and 67 DAS in the treatment of 10 µg P g−1 , but the citrate exudation rates remained unchanged with time for the plants fed with 200 µg P g−1 from 40 to 76 DAS.Figure 5. Concentrations of excess cations in shoots and roots of white lupin grown at 10 or 200 µg P g−1 soil during 76 d. Vertical bars on symbols represent means ±SE (n = 3 replicate pots). The separate bar represents LSD (P = 0.05) for any two means according to an analysis of variance for different treatments.Discussion Effect of P limitation on plant growth and P uptake In the present study, P deficiency led to a significant decrease in biomass of both shoots and roots, but the shoot growth appeared to be more sensitive to P deficiency than root growth. The decrease in plant growth due to P deficiency was also observed in the reduced75 laas et al., 2003). From the first to final harvests, the ratios between P uptake and P adding are 25–50% for 10 µg P g−1 , and 1.7–7.2% for 200 µg P g−1 . However, because P has a low diffusion in soil, particularly FePO4 , only the P close to root surface has a chance to be absorbed. According to the ratio of 10% [generally, 1–5% under field condition (Marschner, 1995)] between root volume and total soil volume, only 3 mg and 60 mg FePO4 can be readily accessed by plants, and then the ratios between P uptake and the accessed FePO4 become 250–500% and 17–72%, suggesting that much P could be mobilized from soil. The significant difference in P concentrations in plant tissues between P treatments occurred prior to plant fresh weights. The reduced growth of white lupin receiving 10 µg P g−1 was correlated with the decrease in P concentrations in shoots, but had no significant correlation with P concentrations in roots. The results showed that the P concentrations in shoots were not completely dependent on the P concentrations in roots, being consistent with the previous report (Marschner, 1995). Root-induced acidification and excess cation uptake The decreased pH of root exudate solution showed that the low P supply increased H+ release from roots. In symbiotically-grown legumes, rhizosphere acidification may be caused by cation-anion balance, the excretion of organic anions and symbiotic nitrogen fixation (Marschner, 1995; Tang et al., 1997). Under the specific experimental condition, it is not possible to separate the quantitative contribution of each of these processes to total net-release of protons. Moreover, in the present study, all process occurring in soil could have influenced the pH of the leachate and in fact we can not separate the net H+ release from other soil processes. However, the difference in pH between P treatments showed a clear effect of P limitation on H+ release from roots because P limitation caused excess uptake of cations, resulting in much H+ release from roots. Plant roots extrude a net amount of H+ to maintain charge balance when cation uptake exceeds anion uptake (Dinkelaker et al., 1989; Hedley et al., 1982; Marschner, 1995; Tang et al., 2001). In particular, excess cation uptake by plants reflected H+ release when N was supplied through N2 -fixation (Tang et al., 1997). In the present experiment with N2 -fixing Lupinus albus grown in P-deficient soil, net H+ release was most likely caused by excess cation uptake. Phosphorus deficiency increased Ca concen-Figure 6. The concentrations of citrate [µmol (g FW)−−1 ] in shoot and root tissues and citrate exudation [µmol h−1 (g FW)−−1 ] from roots of white lupin grown with 10 and 200 µg P g−1 soil at different growth stages. Vertical bars on symbols represent means ±SE (n = 3 replicate pots). The separate bar represents LSD (P = 0.05) for any two means according to an analysis of variance for different treatments.plant height and number of flowers per plant after 58 DAS. The reduced fresh weight and plant height were the first visible symptoms of P deficiency. The increased fresh weights and P contents in the adequate P plants in comparison to the limited P plants showed that white lupin plants could take up large amounts of P from FePO4 added in soil, and that the amount of P taken up increased with the amounts of FePO4 added in soil despite FePO4 being a form of sparingly soluble P. The results showed a support for the viewpoint that white lupin plants have a great ability of mobilizing the sparingly soluble P through changing rhizosphere processes, particularly, citrate exudation (Hinsinger, 1998; Marschner, 1995; Venek-76 trations in shoots and roots, and Mg concentrations in shoots, but decreased S accumulation in shoots, showing that concentrations of excess cations was higher in the P-deficient than P-sufficient N2 -fixing plants. Furthermore, a clear negative correlation was observed between the number of cluster roots (Shen et al., 2003) and the pH of root exudate solution, suggesting that cluster roots of white lupin are the main site for release of H+ under P deficiency, which is consistent with other studies (Dinkelaker et al., 1989, 1995; Marschner, 1995; Neumann and Römheld, 1999; Watt and Evans, 1999a; Yan et al., 2002). Relationship between proton release, accumulation and exudation of carboxylates Accumulation of citrate was found in shoot and root tissues of P-deficient plants, which is in agreement with the observation of citrate accumulation in mature cluster roots under P deficiency (Neumann et al., 1999). However, in the present study, concentrations of citrate in shoots and roots of the plants fed with 10 µg P g−1 soil increased continuously with time (Figure 6), while a higher exudation of citrate was observed at 49 and 67 DAS in the treatment of 10 µg P g−1 (Figure 6 calculated from Shen et al., 2003). These results showed that the high concentrations of citrate in plants could be a prerequisite for citrate exudation, but citrate exudation did not show the consistent change pattern with time in comparison to citrate accumulation in plant tissue, being consistent with previous reports (Keerthisinghe et al., 1998; Neumann et al., 1999). Moreover, exudation of citrate showed a clearly different pattern of change with time compared with the pH of root exudate solution, showing a lack of dependence of proton release on exudation of citrate anions. The results suggest that exudation of citrate contributes only a part of total acidification and excess cation uptake is the dominant factor affecting net proton release from roots of white lupin plants grown in P-deficient soil. In conclusion, white lupin plants can take up large amounts of P from FePO4 as a sparingly soluble P form through changing rhizosphere processes. Phosphorus deficiency of N2 -fixing plants grown in soil resulted in excess cation uptake and enhanced concomitant proton release, causing a decreased pH of root exudate solution. Citrate exudation did not show the consistent change pattern with time in comparison to citrate accumulation in plant tissue. Exudation of citrate anions contributes only a part of total acidification, but excess cation uptake dominantly contributes net proton release from roots of white lupin plants grown in P-deficient soil. Acknowledgements This study was supported by grants from the MSBRDP (Project No: G1999011709), by the National Natural Science Foundation of China (No. 30000102) and by AUSAid. ReferencesBarber S A 1984 Soil Nutrient Bioavailability: A mechanistic approach. John Wiley & Sons, Inc. New York. 398 p. Brennan R F, Gartrell J W and Robson A D 1980 Reactions of copper with soil affecting its availability to plants. I. Effect of soil type and time. Aust. J. Soil Res. 18, 447–459. Dinkelaker B, Romheld V and Marschner H 1989 Citric acid excretion and precipitation of calcium in the rhizosphere of white lupin (Lupinus albus L.). Plant Cell Environ. 12, 285–292. Dinkelaker B, Hengeler C and Marschner H 1995 Distribution and function of proteoid roots and other root clusters. Bot. Acta. 108, 183–200. Dracup M N H, Barrett-Lennard E G, Greenway H and Robson A D 1984 Effect of phosphorus deficiency on phosphatase activity of cell walls from roots of subterranean clover. J. Exp. Bot. 35, 466–480. Föhse D, Classen N and Jungk A 1991 Phosphorus efficiency of plants. II. Significance of root radius, root hairs and cation-anion balance for phosphorus influx in seven plant species. Plant Soil 132, 261–272. Gardner W K, Barber D A and Parbery K G 1983 The acquisition of phosphorus by Lupinus albus L. III. The probable mechanism by which phosphorus movement in the soil/root interface is enhanced. Plant Soil 70, 107–124. Gerke J, Roemer W and Jungk A 1994 The excretion of citric and malic acid by proteoid roots of Lupinus albus L. Effect on soil solution concentrations of phosphate, iron and aluminium in the proteoid rhizosphere in samples of an oxisol and luvisol. Z. Pflanzenernähr. Bodenkd. 157, 289–294. Hedley M J, White R E and Nye P H 1982 Plant-induced changes in the rhizosphere of rape (Brassica napus var. Emerald) seedlings. III. Changes in L value, soil phosphate fractions and phosphatase activity. New Phytol. 91, 45–56. Hinsinger P 1998 How do plant roots acquire mineral nutrients? Chemical properties involved in the rhizosphere. Adv. Agron. 64, 225–265. Hinsinger P 2001 Bioavailability of soil inorganic P in the rhizosphere as affected by root-induced chemical changes: A review. Plant Soil 237, 173–195. Hinsinger P, Plassard C, Tang C and Jaillard B 2003 Origins of rootmediated pH changes in the rhizosphere and their responses to environmental constraints: A review. Plant Soil 248, 43–59. Johnson C M and Ulrich A 1959 Analytical methods for use in plant analysis. Cali. Agric. Exp. Stat. Bull. No.766. Johnson J F, Allan D L and Vance C P 1994 Phosphorus stressinduced proteiod roots show altered metabolism in Lupinus albus. Plant Physiol. 104, 657–665.。
GRE阅读3大类题型出题考点和解题思路分析指点
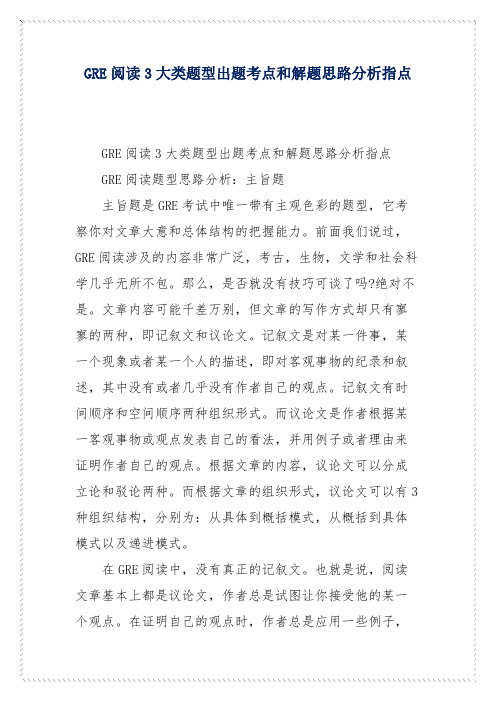
(A) contrast the theories of Jean Wagner with those of other contemporary critics
(B) document the influence of Jean Wagner on the development of Afro-American poetry
GRE阅读题型思路分析:列举题
列举,顾名思义,就是符合某种条件的几个例子,例子可以是某几个事件,动作,情况或者人物。列举题有两种形式,一种是列举非,它问你四个选项中哪一个原文中没有提及。应对的办法仍然是定位原文,然后采用排除法,直至找到正确答案。第二种列举题要你在四个选项种找出哪一个选项在原文中提及,应对的措施仍然是根据关键词定位。其实,列举题并不难,出现的概率也比较大,每篇阅读大概出现一题。
It is Wagner who first demonstrated the essential fusion of racial and religious feeling in Afro-American poetry. The two, he argued, form a symbiotic union in which religious feelings are often applied to racial issues and racial problems are often projected onto a metaphysical plane. Wagner found this most eloquently illustrated in the Black spiritual, where the desire for freedom in this world and the hope for salvation in the next are inextricably intertwined.
Phytoremediation

Case study
- A liquid fertilizer was supplied weekly to the plants - grow time 4 weeks - Adding selected soil amendments - Plants were harvested 1 week after adding amendments - Shoots were washed - Were dried in an oven at 70°C - Analyzed for U by ICP-AES
Case study
Desorption to soil solution
200 fold increase from 1.2 to 240 mg pH was reduced by 0.5 to 1 unit
Case study
Case study
Case study: Results
- B. juncea is the best plant to accumulate U -Citric acid is the best acid to increase the U uptake of plants -U accumulation is associated with a stronger Pb accumulation -Even in one species there are differences in U uptake -For remediation, the soil has to be washed first with acid to desorp from soil particles - Hyperaccumulation is rapid
Interaction between arbuscular mycorrhizal fungi and Trichoderma harzianum

Applied Soil Ecology 47 (2011) 98–105Contents lists available at ScienceDirectApplied SoilEcologyj o u r n a l h o m e p a g e :w w w.e l s e v i e r.c o m /l o c a t e /a p s o ilInteraction between arbuscular mycorrhizal fungi and Trichoderma harzianum under conventional and low input fertilization field condition in melon crops:Growth response and Fusarium wilt biocontrolAinhoa Martínez-Medina ∗,Antonio Roldán,Jose A.PascualDepartment of Soil and Water Conservation and Organic Waste Management,CSIC-Centro de Edafología y Biología Aplicada del Segura.Campus Universitario de Espinardo,E-30100Espinardo,Murcia,Spaina r t i c l e i n f o Article history:Received 16April 2010Received in revised form 12November 2010Accepted 17November 2010Keywords:Biocontrol FertilizerFusarium oxysporum Glomus sp.MelonTrichoderma harzianuma b s t r a c tThe objective of this work was to evaluate the interactions between four arbuscular mycorrhizal fungi (AMF)(Glomus intraradices ,Glomus mosseae ,Glomus claroideum ,and Glomus constrictum )and the ben-eficial fungus Trichoderma harzianum ,inoculated in a greenhouse nursery,with regard to their effects on melon crop growth under conventional and integrated-system field conditions,and the biocontrol effect against Fusarium wilt.A synergistic effect on AM root colonization due to the interaction between T.harzianum and G.constrictum or G.intraradices ,was observed under a reduced fertilizer dosage,while no significant effect was observed for G.claroideum or G.mosseae.With the reduced fertilizer input,AMF-inoculated plants and T.harzianum -inoculated plants had improved shoot weight and nutritional status,but the combined inoculation of AMF and T.harzianum did not result in an additive effect.Under the con-ventional fertilizer dosage,plant growth was not influenced by AM formation;however,it was increased significantly in T.harzianum -inoculated plants.The AMF-inoculated plants were effective in controlling Fusarium wilt,G.mosseae -inoculated plants showing the greatest capacity for reduction of disease inci-dence.The T.harzianum -inoculated plants were more effective than AMF-inoculated plants with regard to suppressing disease incidence.Co-inoculation of plants with the AMF and T.harzianum produced a more effective control of Fusarium wilt than each AMF inoculated alone,but with an effectiveness similar to that of T.harzianum -inoculated plants.© 2010 Elsevier B.V. All rights reserved.1.IntroductionIn recent years,low-input agricultural systems have gained increasing importance in many industrialized countries,for reduc-tion of environmental degradation (Mäder et al.,2002).Integrated farming systems with reduced inputs of fertilizers and pesticides have been developed.It is under these conditions that plants are expected to be particularly dependent on beneficial rhizosphere microorganisms (Smith et al.,1997).Arbuscular mycorrhizal fungi (AMF)are key components of soil microbiota and form symbiotic relationships with the roots of most terrestrial plants,improving the nutritional status of their host and protecting it against several soil-borne plant pathogens (Smith et al.,1997;Harrison,1999;Bi et al.,2007).The incidence and the effect of root colonization vary depending on the plant species and the AMF (Jeffries and Barea,2001);they are influenced by soil microorganisms and environmental factors (Azcón-Aguilar∗Corresponding author.Tel.:+34968396339;fax:+34968396213.E-mail address:ammedina@cebas.csic.es (A.Martínez-Medina).and Barea,1992;Bowen and Rovira,1999).Trichoderma sp.is a common component of rhizosphere soil and has been reported to suppress a great number of plant diseases (Chet,1987;Harman and Lumsden,1990;De Meyer et al.,1998;Elad,2000;Howell,2003).Some strains,also,have been reported to colonize the root sur-face,enhancing root growth and development,crop productivity,resistance to abiotic stresses,and the uptake and use of nutrients (Ousley et al.,1994;Björkman et al.,1998;Harman and Björkman,1998;Rabeendran et al.,2000;Harman et al.,2004).Several reports have demonstrated that the interaction of these two groups of microorganisms may be beneficial for both plant growth and plant disease control (Linderman,1992;Barea et al.,1997;Saldajeno et al.,2008;Martínez-Medina et al.,2009a ).A syn-ergistic effect of some saprophytic fungi on AMF spore germination and colonization has been confirmed (Calvet et al.,1993;McAllister et al.,1996;Fracchia et al.,1998).For example,it has been reported that some Trichoderma strains may influence AMF activity (Calvet et al.,1992,1993;Brimner and Boland,2003;Martinez et al.,2004;Martínez-Medina et al.,2009a ).Volatile and soluble exudates pro-duced by saprophytic fungi are involved in these effects (McAllister et al.,1994,1995;Fracchia et al.,1998).Nevertheless,the results0929-1393/$–see front matter © 2010 Elsevier B.V. All rights reserved.doi:10.1016/j.apsoil.2010.11.010A.Martínez-Medina et al./Applied Soil Ecology47 (2011) 98–10599of research on the interactions between soil saprophytic and AM fungi differ widely,even when the same species of saprophytic fungi are involved.For example,Trichoderma harzianum has been found to have antagonistic,neutral,and stimulating effects on AMF (Rousseau et al.,1996;Siddiqui and Mahmood,1996;Fracchia et al., 1998;Godeas et al.,1999;Green et al.,1999;Martínez-Medina et al., 2009a).Little is known about the interactions between AMF and beneficial saprophytic fungi,and the few studies published on this topic do not provide any conclusivefindings(Green et al.,1999; Vázquez et al.,2000).Even more,the beneficial effect attributable to these interactions under controlled experimental conditions may not be reflected infield experiments(Calvet et al.,1992;McAllister et al.,1997;Fracchia et al.,1998;Vázquez et al.,2000;Martinez et al.,2004).The aim of this work was to evaluate the effect of T.harzianum and four AMF,previously inoculated in a greenhouse nursery, with regard to melon plant growth and their potential biocontrol of Fusarium wilt,under different soil fertilization conditions.To achieve this aim,dual inoculation in a greenhouse nursery with four mycorrhizal fungi from the genus Glomus(G.constrictum,G. mosseae,G.claroideum and Glomus intraradices)and the fungus T. harzianum was evaluated in twofield experiments(under conven-tional conditions and with reduced fertilizer dosage,and under F. oxysporum pressure)for its effect on melon crops,with regard to(1)plant growth and(2)biocontrol of Fusarium wilt.2.Material and methods2.1.Host plant and fungal inoculaMelon plants(Cucumis melo L.,cv.“Giotto”)were used as the host plants.Plants were inoculated with T.harzianum and four dif-ferent AMF from the genus Glomus(G.constrictum,G.mosseae,G. claroideum,and G.intraradices)in a greenhouse nursery(Martínez-Medina et al.,2009a).Here,the AM inocula were mixed at a rate of20g kg−1of peat,while T.harzianum was added to reach a population density of1×106conidia g−1of peat,according to Martínez-Medina et al.(2009a).The AM fungal inoculum density was found to be35infective propagules per gram of inoculum. The isolate of T.harzianum,deposited in the Spanish Type Culture Collection(isolate CECT20714)by Centro de Edafología y Biologia Aplicada del Segura-CSIC(Spain),was chosen for this study owed to its high biocontrol capacity against F.oxysporum(Martínez-Medina et al.,2009a).T.harzianum inoculum was produced using a specific solid medium,prepared by mixing commercial oats,bentonite and vermiculite(1:2.5:5,w:v:v)according to Martínez-Medina et al. (2009b).The plants were grown in a peat-vermiculite mixture under nat-ural conditions,forfive weeks.They were irrigated manually,as necessary,during this period.Five weeks after planting,the melon plants were transplanted to thefield,at the Estación Experimen-tal Cuatro Caminos(Spain)(38◦11 N;1◦03 W),where they were arranged in a randomized design.Monoconidial Fusarium oxysporum f.sp.melonis was isolated from infected melon plants from a greenhouse nursery.For the production of inocula,the pathogen was cultivated for5days on potato dextrose broth(Scharlau Chemie,Barcelona,Spain),at28◦C in darkness,on a shaker at120rpm.After the incubation period,the fungal culture was centrifuged at193×g,10min,re-suspended in sterilized water,and re-centrifuged.The fungal suspension con-tained1×108conidia mL−1.2.2.Experimental design and growth conditionsTwo experiments,using a completely randomized design,were conducted separately.Thefirst experiment had three factors,thefirst factor withfive levels:non-inoculation and inoculation with four AMF(G.constrictum,G.mosseae,G.claroideum,or G. intraradices)in the greenhouse nursery.The second factor had two levels:non-inoculation and inoculation with T.harzianum,in the greenhouse nursery.The third factor had two levels:conventional and reduced fertilization dosage.To assess the effect of the fertilization on the interactions between the AMF and T.harzianum,half of the experiment was fertirrigated with a conventional fertilization dose for melon plants in the Mediterranean area:0.51g L−1NH4NO3and0.51g L−1 NH4H2PO4.The other half of the experiment was fertirrigated with 1/3of this dose.Eight replicates were established for each of the20 treatments.The second experiment had three factors,thefirst factor with five levels:non-inoculation and inoculation in the greenhouse nursery with four AMF(G.constrictum,G.mosseae,G.claroideum,or G.intraradices).The second factor had two levels:non-inoculation and inoculation in the greenhouse nursery with T.harzianum.The third factor had two levels:non-inoculation and inoculation with F.oxysporum.To assess the effect of the interactions between the AMF and T.harzianum on the potential biocontrol of Fusarium wilt, four weeks after planting,half of the melon plants were infected by F.oxysporum to reach afinal concentration of1×104conidia g−1 in the rhizosphere,while the other half was maintained as a control.Eight replicates were established for each of the20treat-ments.For both experiments,plants were planted1m apart,at a depth of10cm,in rows.The soil had a pH of8.04(1:1soil:water ratio), the NaHCO3-extractable P was26g g−1,total N was1mg g−1, and extractable K was289g g−1.The soil texture was39g kg−1 coarse sand,502g kg−1fine sand,301g kg−1silt,and158g kg−1 clay.Plants were grown for eleven weeks in thefield under natural conditions(the climate is semi-arid Mediterranean with an aver-age annual rainfall of300mm and a mean annual temperature of 19.2◦C;the potential evapo-transpiration reaches1000mm/year). Plants were fertirrigated automatically for10min every12h with 2.5L h−1water drippers.The fertilizer was added in fertigation at the following doses:0.13g L−1NH4NO3(total nitrogen:35.5%, nitric nitrogen:16.9%,ammoniacal nitrogen:17.6%)and0.13g L−1 NH4H2PO4(ammoniacal nitrogen:12%,soluble P2O5in neutral ammonium citrate:60%).Eleven weeks after planting,plants were harvested and rhi-zosphere samples were taken and stored at4◦C for biological and biochemical analyses.In thefirst experiment,the shoot fresh weight and the nitrogen,phosphorus,and potassium contents were recorded as well as the fruit production.In the second experiment, further F.oxysporum-infected plants were determined.2.3.Plant analysesPlant samples for nutrient content analysis were digested by a microwave technique,using a Milestone Ethos I microwave diges-tion instrument.A standard aliquot(0.1g)of dry,finely ground plant material was digested with concentrated nitric acid(HNO3) (8mL)and hydrogen peroxide(H2O2)(2mL).Subsequently,the phosphorus and potassium contents were analyzed using ICP(Iris intrepid II XD2Thermo).Plant nitrogen content was determined using a Flash1112series EA carbon/nitrogen analyzer.Roots were softened with10%KOH in water bath and stained with0.05%trypan blue(Phillips and Hayman,1970).The per-centage root length colonized by AMF was calculated by the line intersect method(Giovannetti and Mosse,1980).Positive counts for AM colonization included the presence of vesicles,arbuscules, or typical mycelium within the roots.To determine the F.oxysporum colonization of inoculated plants, stem segments(∼1.5cm)from inoculated plants were cut imme-100 A.Martínez-Medina et al./Applied Soil Ecology47 (2011) 98–105 diately above crowns,surface-sterilized by soaking in1%sodiumhypochlorite for5min,and rinsed with sterilized water.Thesegments were incubated on PDA at28◦C for6days,and theappearance of F.oxysporum colonies was considered to be indica-tive of infected plants.The percentage of infected plants was usedto determine the disease incidence.2.4.Soil biological analysesSerial dilutions of1g of rhizosphere soil from the top0.3m,insterile,quarter-strength Ringer solution,were used for quantify-ing the T.harzianum colony forming units(CFU)by a plate counttechnique using PDA(Scharlau Chemie,Barcelona,Spain)amendedwith50mg L−1rose bengale and100mg L−1streptomycin sulfate.The plates were incubated at28◦C for5days.After the incubationperiod,CFUs were counted.Komada medium(Komada,1975)wasused for quantification of F.oxysporum.2.5.Statistical analysisThe data were subjected to analysis of variance(ANOVA)usingSPSS software(SPSS system for Windows,version15.0,SPSS Inc,Chicago,II).The statistical significance of the results was deter-mined by performing Duncan’s multiple-range test(P<0.05).3.Results3.1.Experiment I3.1.1.Plant shoot fresh weightIn treatments involving reduced fertilization,plants inoculatedwith T.harzianum alone had significantly increased shoot freshweight relative to the non-inoculated plants(Table1).The AMF-inoculated plants also showed increases in fresh weight,with nodifferences among the AMF.Plants co-inoculated with T.harzianumand AMF showed fresh weights similar to those of AMF-inoculatedplants.Conventionally fertilized plants had a higher fresh weightthan plants receiving a reduced dosage of fertilizer,the factor fer-tilization being highly significant(P<0.001)(Tables1and4).T.harzianum-inoculated plants receiving the conventional fertilizerdosage had an increased fresh weight compared with the non-inoculated plants,while the fresh weight of AMF-inoculated plantsdid not differ from that of the non-inoculated plants.Co-inoculated(T.harzianum-AMF)plants which were fertilized conventionallyexhibited fresh weights similar to those of AMF-inoculated plants(Table1).Table1Fresh shoot weight(g)of plants inoculated or not with Trichoderma harzianumand/or Glomus constrictum,Glomus mosseae,Glomus claroideum,or Glomusintraradices,under conventional and reduced fertilization dosages.Treatment Reduced fertilizerdose Conventional fertilizer doseNon-inoculated772±28c1156±27cG.constrictum890±64b1160±22cG.mosseae854±69b1291±56abc G.claroideum881±22b1154±45cG.intraradices884±47b1257±19abc T.harzianum1057±35a1378±80abG.constrictum×T.harzianum885±9b1166±77cG.mosseae×T.harzianum978±42ab1307±75abc G.claroideum×T.harzianum818±19b1233±121bc G.intraradices×T.harzianum911±14ab1288±55abcData are means±standard error of eight replicates.Values in the same column with the same letters,represent no significant difference between treatments according to Duncan’s multiple range test(P≤0.05),n=8.3.1.2.Nutrient contentWith the low fertilizer dosage,total plant nitrogen was increased(P<0.001)in AMF-inoculated plants(Tables2and4). Inoculation of plants with T.harzianum increased the nitrogen content significantly.No additive effect for nitrogen content was observed in plants co-inoculated with T.harzianum and AMF;even negative effect could be observed in the case of plants co-inoculated with G.constrictum and T.harzianum.The plants co-inoculated with T.harzianum and G.mosseae showed higher nitrogen contents than plants co-inoculated with any other AMF.The conventional fer-tilization dose increased the plant nitrogen concentration in all treatments(P<0.001)(Tables3and4).The nitrogen content was increased in T.harzianum-inoculated plants,while no differences in nitrogen content were found in AMF-inoculated plants,compared to the non-inoculated plants.Co-inoculation with T.harzianum and AMF gave nitrogen values similar to those of plants inoculated with the AMF alone.Under reduced fertilizer dosage,the phosphorus concentration was increased(P<0.001)in plants which had been inoculated in the greenhouse nursery with G.mosseae,G.claroideum,or G.intraradices alone,but it was unaffected in G.constrictum-inoculated plants(Tables2and4).The phosphorus content of T.harzianum-inoculated plants at the reduced fertilizer dosage was increased with respect to the non-inoculated plants.The plants co-inoculated with T.harzianum and AMF showed phos-phorus levels similar to those of plants inoculated with the AMF alone.The conventional fertilizer application increased(P<0.001) the shoot phosphorus level in all the treatments compared with the lower dose(Tables3and4).The phosphorus content of T. harzianum-inoculated plants was not altered with respect to non-inoculated plants.A decreased phosphorus level was observed in AMF-inoculated plants,and in plants co-inoculated with AMF and T. harzianum,relative to non-inoculated plants.Co-inoculated plants showed lower phosphorus contents than T.harzianum-inoculated plants.With the low fertilizer dosage,the plant potassium con-tent was increased in plants which had been inoculated in the greenhouse nursery with the AMF(Table2).The potas-sium content of T.harzianum-inoculated plants was increased with respect to the non-inoculated plants,at the reduced fertil-izer dosage.The potassium contents of plants co-inoculated with T.harzianum and AMF were similar to those of plants inocu-lated with each AMF alone,with no differences among them or with respect to T.harzianum-inoculated plants.The factor fer-tilization was not significant for the plant potassium content (Table4).At the conventional fertilizer dosage,no differences in plant potassium content were found among the treatments (Table3).3.1.3.AM root colonizationInoculation in the greenhouse nursery with the different AMF produced a significant increase(P<0.001)in the AM root coloniza-tion underfield conditions(Fig.1).Under low fertilizer dosage,G. constrictum-and G.intraradices-inoculated plants showed higher percentages of AM root colonization than any other AMF tested. The lowest percentage of AM colonization was observed in G. claroideum-inoculated plants.Under the reduced fertilizer dosage, AM root colonization by G.constrictum or G.intraradices was increased(P<0.001)in plants which were also co-inoculated with T.harzianum,with respect to plants inoculated with AMF alone,but it was unaffected in plants co-inoculated with G. mosseae or G.claroideum and T.harzianum.The conventional fer-tilizer dosage produced,in general,a decreased percentage of AM root colonization(P<0.001)compared with the reduced fertilizer dose.A.Martínez-Medina et al./Applied Soil Ecology47 (2011) 98–105101Table2Shoot nitrogen,phosphorus,and potassium contents(g per plant)of melon plants inoculated or not with Trichoderma harzianum and/or Glomus constrictum,Glomus mosseae, Glomus claroideum,or Glomus intraradices,under reduced fertilization dosage.Treatment Nitrogen Phosphorous PotassiumNon-inoculated 1.90±0.01c0.37±0.02c 1.18±0.09cG.constrictum 2.11±0.01b0.36±0.02c 1.56±0.16abG.mosseae 2.49±0.01ab0.62±0.17ab 1.73±0.40aG.claroideum 2.08±0.16b0.47±0.05b 1.51±0.16abG.intraradices 2.05±0.05b0.45±0.02b 1.34±0.21bT.harzianum 2.42±0.06ab0.88±0.09a 1.51±0.13abG.constrictum×T.harzianum 1.82±0.29c0.39±0.09bc 1.52±0.05abG.mosseae×T.harzianum 2.63±0.07a0.48±0.05b 1.66±0.44abG.claroideum×T.harzianum 1.94±0.11bc0.49±0.19b 1.29±0.59bG.intraradices×T.harzianum 2.07±0.23b0.41±0.01bc 1.33±0.46bData are means±standard error of eight replicates.Values in the same column with the same letters,represent no significant difference between treatments according to Duncan’s multiple range test(P≤0.05),n=8.Table3Shoot nitrogen,phosphorus,and potassium contents(g per plant)of melon plants inoculated or not with Trichoderma harzianum and/or Glomus constrictum,Glomus mosseae, Glomus claroideum,or Glomus intraradices,under conventional fertilization dosage.Treatment Nitrogen Phosphorous PotassiumNon-inoculated 4.74±0.01bcd 1.49±0.15a 1.20±0.14abG.constrictum 4.39±0.05bcde 1.11±0.40bc 1.56±0.49abG.mosseae 4.80±0.49bcd 1.16±0.21bc 1.55±0.46abG.claroideum 4.19±0.34cde0.95±0.06c 1.19±0.28abG.intraradices 5.46±0.27ab 1.21±0.09bc 1.70±0.62aT.harzianum 5.72±0.81a 1.59±0.57a 1.78±0.84aG.constrictum×T.harzianum 3.73±0.32de0.98±0.42c 1.00±0.21abG.mosseae×T.harzianum 5.02±0.64abc 1.10±0.36bc 1.47±0.35abG.claroideum×T.harzianum 3.56±0.04e0.99±0.14c0.99±0.03bG.intraradices×T.harzianum 4.98±0.17abc0.93±0.02c 1.77±0.03aData are means±standard error of eight replicates.Values in the same column with the same letters,represent no significant difference between treatments according to Duncan’s multiple range test(P≤0.05),n=8.3.1.4.T.harzianum populationT.harzianum was detected in the rhizosphere,reaching values around1×104CFU g−1and showing similar CFU values in all the treatments which included inoculation with T.harzianum;in non-inoculated treatments,its density was below1×102CFU g−1(data not shown).3.1.5.Number of fruitsWith the reduced fertilizer dosage,AMF-inoculated plants had an increased number of fruits(P<0.01)compared with non-inoculated plants(Fig.2).T.harzianum-inoculated plants did not differ in their fruit number relative to non-inoculated plants. At the reduced fertilizer dose,and compared with the AMF-inoculated plants,the number of fruits was decreased significantly by T.harzianum–G.constrictum or T.harzianum–G.intraradices co-inoculation,whereas T.harzianum–G.mosseae co-inoculation significantly increased the number of fruits.The conventional fertilization dose reduced significantly the number of fruits(P<0.001),compared with the lower dose(Fig.2). No.significant differences in fruit number were produced by AMF or T.harzianum inoculation,alone or in combination,at the con-ventional fertilizer dosage,compared to non-inoculated plants. 3.2.Experiment II3.2.1.T.harzianum populationT.harzianum was detected in the rhizosphere,reaching values around1×104CFU g−1and showing similar CFU values in all the treatments which included inoculation with T.harzianum;in non-inoculated treatments,its density was below1×102CFU g−1(data not shown).3.2.2.Disease incidenceThe disease incidence in AMF-inoculated plants was reduced by up25–50%,G.mosseae-inoculated plants showing the low-est percentage of infection(Fig.3).The disease incidence in T. harzianum-inoculated plants was reduced by60%with respect to non-inoculated plants.Plants co-inoculated with T.harzianum and AMF showed a lower percentage infection than AMF-inoculated plants.Table4The three-factor ANOVA(arbuscular mycorrhizal fungi(AMF)inoculation,Trichoderma harzianum inoculation,and fertilization(F)level)for all parameters studied.P significant values.Parameters studied AMFinoculation AM T.harzianuminoculation ThFertilization F InteractionAM×ThInteractionAM×FInteractionTh×FInteractionAM×Th×FShoot fresh weight0.0290.008<0.0010.0200.005NS NS Nitrogen content<0.0010.05<0.001<0.001<0.001NS NS Phosphorus content<0.0010.045<0.001NS NS NS NS Potassium content0.030.05NS0.045NS NS NS AM root colonization<0.001NS<0.001<0.001<0.001<0.001NS Fruit number0.0060.027<0.0010.017NS NS NS T.harzianum population NS<0.001NS<0.001NS<0.001NSNS:non-significant.102 A.Martínez-Medina et al./Applied Soil Ecology47 (2011) 98–105Fig.1.Percentage of root length colonized by Glomus constrictum ,Glomus mosseae ,Glomus claroideum ,and Glomus intraradices in melon plants receiving conventional or reduced fertilization and co-inoculated or not with Trichoderma harzianum .Bars indicate standard error of eight replicates.Values with the same letter do not differ significantly according to Duncan’s multiple range test (P ≤0.05),n =8.4.DiscussionThe results show a synergistic effect on AM root colonization due to the interaction between T.harzianum and G.constrictum or G.intraradices ,while no significant effect was observed for G.claroideum and G.mosseae .Although saprophytic fungi have been reported to influence AM colonization and host plant response (Fracchia et al.,2000),the effects of the saprophytic fungi on AM formation differ depending on the inherent characteristic of both agents (Martinez et al.,2004;Saldajeno et al.,2008;Martínez-Medina et al.,2009a ).A synergistic interaction between T.aureoviride and G.mosseae has been reported for AM root col-onization (Calvet et al.,1993).Fracchia et al.(1998)found that T.harzianum did not affect the percentage of soybean root length col-onized by G.mosseae ,whereas T.pseudokoningii increased it.Calvet et al.(1992)reported a stimulation of G.mosseae spore germination by T.harzianum and T.aureoviride .The synergistic effect produced by the interaction between T.harzianum and G.constrictum or G.intraradices in our experiment could have been caused by a direct beneficial action of soluble exudates and volatile compounds pro-duced by the saprophytic fungus (Calvet et al.,1992).No negative interaction was observed in our results,in contrast to previous results (McAllister et al.,1996;Green et al.,1999;Martinez et al.,2004).Our results further demonstrate that,under reduced fertilizer dosage,AMF and T.harzianum inoculation resulted in an improve-ment in shoot weight and nutritional status.Soil microorganisms and their activities play important roles in thetransformationFig.2.Number of fruits produced by plants inoculated with Trichoderma harzianum and/or Glomus constrictum ,Glomus mosseae ,Glomus claroideum ,or Glomus intraradices ,under conventional and reduced fertilization doses.Bars indicate standard error of eight replicates.Values with the same letter do not differ significantly according to Duncan’s multiple range test (P ≤0.05),n =8.A.Martínez-Medina et al./Applied Soil Ecology47 (2011) 98–105103Fig.3.Disease incidence(%)in plants inoculated with Trichoderma harzianum and/or Glomus constrictum,Glomus mosseae,Glomus claroideum,or Glomus intraradices, seven weeks after pathogen inoculation.Bars indicate standard error of eight repli-cates.Values with the same letter do not differ significantly according to Duncan’s multiple range test(P≤0.05),n=8.of plant nutrients from unavailable to available forms and the improvement of soil fertility(Adesemoye and Kloepper,2009).The capacity of AMF to promote plant growth and enhance phospho-rous availability and uptake has been widely reported over the years(Ames et al.,1983;Smith et al.,1997;Barea et al.,2002; Tawaraya et al.,2006).Several investigations indicated as well,that plant interaction with Trichoderma sp.correlates with improved phosphorous availability and plant growth(Harman and Björkman, 1998;Altomare et al.,1999).However,the combined inoculation of AMF and T.harzianum did not result in an additive effect.In general, for co-inoculated plants,both growth and nutrient uptake were maintained at values similar to those of plants inoculated with the AMF alone.In contrast to our results,Haggag and Abd-El latif(2001) found that the combined inoculation of G.mosseae and T.harzianum enhanced growth of geranium plants.Similarly,combined inocu-lation of T.aureoviride and G.mosseae had a synergistic effect on the growth of marigold plants(Calvet et al.,1993).However,root and shoot weights of soybean were decreased by co-inoculation with T.pseudokoningii and Gigaspora rosea(Martinez et al.,2004). The interaction between AMF and T.harzianum and its effect on plant growth may vary depending on the inherent characteristics of the AMF and the T.harzianum strain(Saldajeno et al.,2008).In our experiment,this interaction was in fact negative in the case of plants co-inoculated with G.constrictum and T.harzianum,which showed a decrease in nitrogen content relative to plants inocu-lated with the AMF or T.harzianum alone.However,an increase in the plant nitrogen content was observed in plants which had been co-inoculated with T.harzianum and G.mosseae in the greenhouse nursery,relative to plants inoculated with the saprophyte alone. Co-inoculation with T.harzianum and G.mosseae was more effec-tive than any other combination tested with regard to increases in the uptake of nitrogen.Under the conventional fertilizer dose,plant growth was not influenced by AM formation,but it was significantly increased when T.harzianum was inoculated alone.However,the growth pro-motion mediated by T.harzianum was decreased at this fertilizer rate.Rabeendran et al.(2000)hypothesized that when plants are grown under optimal conditions growth promotion by Trichoderma is unlikely,whereas under suboptimal conditions enhanced growth can be achieved.Our results show that differences in growth pro-motion by T.harzianum and AMF are related to differences in growing conditions,being more pronounced in soils relatively poor in nutrients.It is noteworthy that plants co-inoculated with the AMF and T.harzianum had growth which was similar to that of non-inoculated plants under these conditions.The negative impact of high N and P levels on mycorrhizal root colonization has been reported(Rubio et al.,2002;Kohler et al.,2006).In our experiment,under the higher fertilization dose, the beneficial effect of the AMF disappeared and the effect was even negative in the case of phosphorus uptake.The suppression of extraradical mycelium development,which occurs in soil fol-lowing a high fertilizer application(Azcón et al.,2003),could not explain ourfindings,since under this condition no effect on plant growth should be expected.This negative effect may be explained by an alteration in the rhizosphere microbial population due to the nutrient supply(Liu et al.,2000;Rengel and Marschner,2005). Stimulation of the rhizospheric population may increase competi-tion between plant roots and the microbial population,which has particular nutrient requirements(Germida et al.,1998;Griffiths et al.,1999),microorganisms being,in many cases,superior com-petitors(Kaye and Hart,1997;Hodge et al.,2000).Similar results have been reported by Azcón et al.(2003),who observed that a higher application of nitrogen and phosphorus to the soil reduced the nutrient uptake in AM-compared with non-AM-lettuce plants. Ourfindings may indicate not only the lack of mycorrhizal ben-efit at these high fertilizer doses,but also a negative influence of AMF on mechanisms associated with the mineral nutrition of plants when grown in a highly fertilized soil.These results suggest that the beneficial mycorrhizal effect on plant nutrition is only evident under lower fertility levels and that fertilizer application can reduce or even eliminate it.Interestingly,a decrease in fruit number was observed due to an increase in the fertilizer dose.Imbalanced fer-tilizer use in soil has been reported to cause yield decline(Manna et al.,2005).In our experiment,T.harzianum was able to increase plant nitrogen uptake even at the higher fertilizer level.Ourfind-ings indicate an improvement in plant active-uptake mechanisms, and an increase in the effectiveness of nitrogen-containing fertil-izer.These results contrast markedly with the absence of effects observed in tomato plants under fertilized conditions:no effects on plant nutritional status occurred following inoculation with Tri-choderma(Inbar et al.,1994).The AMF-inoculated plants showed a significant decrease in Fusarium wilt incidence,G.mosseae-inoculated plants showing the greatest reduction.AM symbiosis has been shown to reduce the damage caused by soil-borne plant pathogens(Azcón-Aguilar and Barea,1996;Bi et al.,2007).Com-petition for host photosynthates or sites,microbial changes in the mycorrhizosphere due to AM,and induction of local and systemic defense responses have been proposed(Azcón-Aguilar and Bago, 1994;Caron,1989;Liu et al.,2007).With regard to suppress-ing disease incidence,T.harzianum was more effective than the AMF.Several studies report the biocontrol capacity of Trichoderma sp.(Chet,1987;Chet et al.,1997;De Meyer et al.,1998;Yedidia et al.,1999;Harman,2000;Howell et al.,2000;Yedidia et al., 2003;Shoresh et al.,2005;Martínez-Medina et al.,2009b).Various mechanisms of biocontrol have been reported,such as mycopara-sitism,antibiotic production,competition,or induction of local and systemic defense responses(Howell,2003;Yedidia et al.,2003; Harman et al.,2004).Co-inoculated plants showed disease sup-pression similar to that of T.harzianum-inoculated plants.Datnoff et al.(1995)reported a higher suppressive effect against Fusarium crown and root rot of tomato with the combination of T.harzianum and G.intraradices than with each biological agent applied alone. However,there are several examples of combinations of different biocontrol agents providing no better or,in some cases,worse bio-control than the isolates used singly(Larkin and Fravel,1998;de Boer et al.,1999).。
Critical Reviews in Oral Biology & Medicine
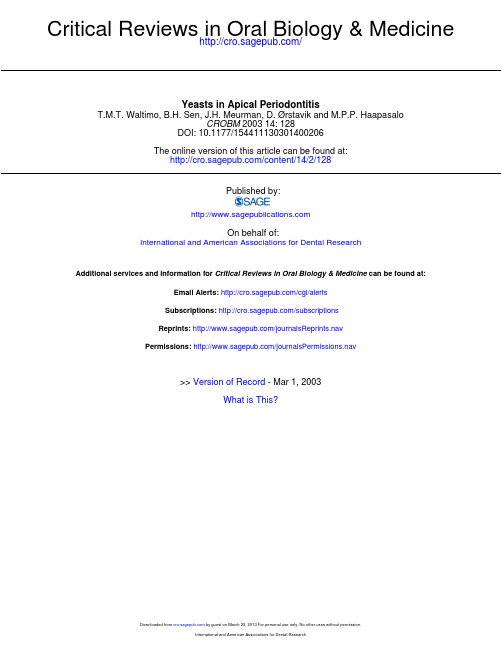
/Critical Reviews in Oral Biology & Medicine/content/14/2/128The online version of this article can be found at:DOI: 10.1177/1544111303014002062003 14: 128CROBM T.M.T. Waltimo, B.H. Sen, J.H. Meurman, D. Ørstavik and M.P.P. HaapasaloYeasts in Apical PeriodontitisPublished by: On behalf of:International and American Associations for Dental Research can be found at:Critical Reviews in Oral Biology & Medicine Additional services and information for/cgi/alerts Email Alerts:/subscriptions Subscriptions: /journalsReprints.nav Reprints:/journalsPermissions.nav Permissions:What is This?- Mar 1, 2003Version of Record >>IntroductionA pical periodontitis is an inflammatory process of the peri-apical area caused by an infection of the dental root canal system. In most cases, chemo-mechanical preparation of the root canal and local medication with calcium hydroxide fol-lowed by filling of the root canal with gutta-percha and sealer result in elimination of the infection and healing of the lesion. Occasionally, apical periodontitis does not respond favorably to root canal therapy, and periapical inflammation caused by the root canal infection may persist months or even years despite treatment. Several factors may contribute to the failure of the treatment of persistent cases. Most commonly, these fac-tors are related to difficulties in chemo-mechanical prepara-tion of the root canals. Occasionally, micro-organisms resistant to conservative therapy may also be involved (Byström, 1986; Sirén et al., 1997). Literature on the microbiological findings in persistent apical periodontitis not responding favorably to conservative therapy is limited (Bender and Selzer, 1952; Grahnen and K rasse, 1963; Engström, 1964; Goldman and Pearson, 1969; Haapasalo et al., 1983; Ranta et al., 1988; Sirén et al., 1997; Molander et al., 1998; Hancock et al., 2001; Kalfas et al., 2001; Love, 2001). However, it is known that a few species are more frequently isolated from persistent cases compared with primary cases. These include the Enterococcus faecalis/faecium group, enteric Gram-negative facultative rods, i.e., coliforms, and Pseudomonas species (Engström, 1964; Haapasalo et al., 1983; Ranta et al., 1988; Sirén et al., 1997; Hancock et al., 2001;Love, 2001). Recently, there has also been increasing interest in the presence and role of yeasts in infections resistant to con-servative root canal therapy (Nair et al., 1990; Sen et al., 1995, 1997a,b). This review presents the contemporary knowledge of the occurrence and biotypes of yeasts in endodontic infections, and susceptibility, in vitro, of yeasts to endodontic irrigants, local disinfectants, and antifungal agents.Taxonomy and General Characteristics of Yeasts Yeasts belong to a separate kingdom of living organisms, fungi. Contrary to bacteria, fungi are eukaryotic organisms, i.e., their genome is organized in a nucleus which is surrounded by a membrane. This membrane is continuous with the endoplas-mic reticulum, and organelles, such as mitochondria, ribo-somes, and different storage inclusions, are present. Fungal cell walls are rigid structures composed mainly of glucan, mannan, and chitin. For nutrition, fungal organisms are dependent on nitrogen and carbon compounds which are taken up through the cell wall (De Hoog and Guarro, 1995).Yeasts are present in various sites in the human body as members of the normal flora. They occur, e.g., in the gastro-intestinal tract, vagina, and perineal area (Jarvis, 1996). The oral cavity has suitable environmental conditions for yeast colo-nization. Oral yeasts belong to the division Ascomycota and class Endomycetes, which is divided further into four families: Saccharomycetaceae, Endomycetaceae, Dipodascaceae, and Lipomycetaceae. Clinically, the most important oral yeastsY EASTS IN A PICAL P ERIODONTITIST.M.T. Waltimo1*B.H. Sen2J.H. Meurman3D. Ørstavik4M.P.P. Haapasalo51Institute of Dentistry, University of Turku, Lemminkäisenkatu 2, 20520 Turku, Finland; 2Department of Restorative Dentistry and Endodontics, Ege University, Izmir, Turkey; 3Institute of Dentistry, University of Helsinki, and Department of Oral and Maxillofacial Diseases, Helsinki University Central Hospital, Finland; 4NIOM, Scandinavian Institute of Dental Materials, Haslum, Norway; and 5Department of Endodontics, Dental Faculty, University of Oslo, Norway; *corresponding author, tuomas.waltimo@utu.fiABSTRACT: Microbiological reports of apical periodontitis have revealed that yeasts can be isolated from approximately 5-20% of infected root canals. They occur either in pure cultures or together with bacteria. Almost all isolated yeasts belong to the genus Candida, and the predominant species is C. albicans. Pheno- and genotypic profiles of C. albicans isolates show hetero-geneity comparable with those of isolates from other oral sites. C. albicans expresses several virulence factors that are capable of infecting the dentin-pulp complex, including dentinal tubules. This causes, consequentially, an inflammatory response around the root apex, which suggests a pathogenic role for this organism in apical periodontitis. Yeasts are particularly associated with persistent root canal infections that do not respond favorably to conservative root canal therapy. This may be due to the resis-tance of all oral Candida species against a commonly used topical medicament, calcium hydroxide. However, other antimicro-bial agents may offer alternative therapeutic approaches and improve the treatment of these persistent cases of apical perio-dontitis.Key words.Apical periodontitis, Candida, endodontics, yeast infection.128Crit Rev Oral Biol Med14(2):128-137(2003)belong to the family Saccharomycetaceae and to the genus Candida . Reproduction of Candida is based on multilateral bud-ding, which may take place anywhere on the mother cell (de Hoog and Guarro, 1995).Oral Yeast SpeciesCandida albicans is the most dominant oral yeast species, fol-lowed by C. glabrata, C. krusei, C. tropicalis, C. guilliermondii, C.kefyr, and C. parapsilosis (Odds, 1988). Recent findings also sug-gest the occasional occurrence of C. dubliniensis, which is a species closely related to C. albicans (Hannula et al ., 1997). Other yeast genera have also been isolated from the oral normal flora,e.g., Saccharomyces spp. and Geotrichum spp. (Tawfik et al ., 1989;Stenderup, 1990; Heinic et al ., 1992). Isolation of other fungi from the oral cavity has also been reported, but they are usual-ly seen in association with systemic disease—for example, pul-monary cryptococcosis caused by Cryptococcus neoformans (Stenderup, 1990).Virulence Factors of CandidaThe transition of C. albicans from a harmless commensal to apathogenic organism appears to be dependent on minorchanges in predisposing conditions which cause the expres-sion of a variety of virulence factors (Shepherd, 1992; Sweet,1997). These factors include adherence, hyphal formation,thigmotropism, protease secretion, and phenotypic switch-ing phenomenon.Adherence of micro-organisms is a complex, multifactori-al process involving several types of cell-surface adhesinswhich are essential for colonization and infection of the host.The main adhesin molecules of C. albicans responsible foradhesion to host cells seem to be cell wall mannoproteins(Sweet, 1997). However, several other factors also contribute tothe adherence of yeasts, e.g., cell-surface hydrophobicity, envi-ronmental pH, and concentrations of iron, calcium, zinc, andcarbon dioxide (Ener and Douglas, 1992; K lotz, 1994;Samaranayake et al ., 1995; Sohnle et al ., 2001). Furthermore,environmental proteins from saliva and gingival crevicularfluid as well as extracellular matrix components affect thecomplex adherence of Candida to host cells and tissues(Calderone et al ., 2000; Holmes et al ., 2002). C. albicans is a pleo-morphic micro-organism demonstrating different growthforms such as germ tubes, yeasts (blastospores), pseudo- andtrue hyphae, and chlamydospores (Odds, 1988; de Hoog andGuarro, 1995). All growth patterns except chlamydosporesmay show conversion to each form of growth, depending onthe environmental conditions. Therefore, the term 'dimorphic',often used in the literature, is semantically inaccurate toexplain C. albicans morphogenesis. Although hyphal formation is not a prerequisite for pathogenicity of C.albicans , biopsies of candidal infections often revealhyphal adherence to and penetration through epithe-lial tissues, indicating increased pathogenicity in com-parison with ovoid yeast forms (Sweet, 1997). It seemsthat the hyphal penetration into tissues is enhanced by thigmotropism, i.e., contact sensing by hyphae to find intracellular junctions or microscopic breaks on mucosal surfaces (Sherwood et al ., 1992; Gow et al .,1994; Sweet, 1997). One of the key virulence determi-nants of Candida species is their ability to produce and secrete aspartyl proteases which digest a variety of host proteins. The virulence of these proteases has beendemonstrated with animal experiments showing that the amount of protease is directly comparable with the patho-genicity of the strain (MacDonald and Odds, 1983; K wong-Chung et al ., 1985; Okamoto et al ., 1993; Togni et al ., 1994).Therefore, the higher rate of protease activity of C. albicans in comparison with other Candida species also suggests higher virulence. In addition to these major virulence factors, C. albi-cans has a tendency to phenotypic alteration, which con-tributes to environmental adaptation. Phenotypic alterations include change of colony morphology and protease activity (Slutsky et al ., 1985; White and Agabian, 1995). This genetical-ly controlled phenomenon is known as phenotypic switching,and it may occur relatively frequently, especially under stress (Soll, 1988). Phenotypic switching may assist in survival of and colonization by the yeasts, and it may also lead to genetic selection of adaptive strains (Sweet, 1997). Virulence factors and their possible contributions to apical periodontitis are list-ed in Table 1.Oral Yeast Infections A characteristic feature of yeast infections is that they develop when the host provides the environmental conditions and nutrients essential for attachment, growth, and reproduction of fungi. In other words, yeasts are opportunistic pathogens.Thus, local or general predisposing factors are required for yeast infection to develop (Shepherd, 1992). These factors can be classified into four categories: (i) host factors, such as nor-mal and pathological changes in physiological status of the host; (ii) dietary factors, such as carbohydrate-rich diets and vitamin deficiencies; (iii) mechanical factors, such as denture-wearing; and (iv) iatrogenic factors, such as administration of broad-spectrum antibiotics and corticosteroids (Odds, 1988).The clinical form of candidosis is often related to a predispo-sing factor, e.g., acute pseudomembraneous candidosis (thrush) is often associated with natural factors such as immunological and microbiological instability at birth, angu-lar cheilitis with dietary factors, chronic atrophic candidosis (denture-associated stomatitis) with mechanical factors, and acute atrophic candidosis with iatrogenic factors (Lynch,1994). In dental practice, chronic atrophic candidosis (denture stomatitis) is perhaps one of the most frequently encountered oral Candida infections (Wilson, 1998). There has also been an increasing interest in the presence of yeasts in infected perio-dontal pockets and their possible role in the pathology of dif-ferent forms of periodontitis (Slots et al ., 1988; Zambon et al .,1990; Rams and Slots, 1991; Dahlén and Wickström, 1995;Hannula et al ., 1997, 2001).14(2):128-137 (2003)Crit Rev Oral Biol Med129TABLE 1Virulence Factors of C. albicans and Their Possible Contributions to Apical Periodontitis Virulence Factor Possible Contribution to Apical Periodontitis Adherence Colonization of dental hard tissues Hyphal formation Penetration into dentinal tubules Thigmotropism Penetration into dentinal tubulesProtease secretion Survival in conditions with limited nutrient supply Phenotypic alteration Adaptation in ecologically harsh conditionsMicrobiology of Apical PeriodontitisApical periodontitis is a host defense response to infection of necrotic pulp (Miller, 1894;Kronfeld, 1939; Kakehashi et al .,1965). The host has an array of defense mechanisms consisting of several types of inflammato-ry cells, such as polymorphonu-clear leukocytes and lympho-cytes, intercellular messengers,such as cytokines, and chemical weapons such as proteolytic enzymes (Nair, 1997). Despite these defenses, the body cannot eliminate the micro-organisms residing in the necrotic root canal, and therefore the inflam-matory process does not result in healing. The interaction between root canal infection and the host defense mecha-nisms eventually cause destruc-tion of periapical tissues and formation of apical periodonti-tis (Nair, 1997).More than 300 species of micro-organisms colonize the human oral cavity, but only a limited number of these have been isolated from infected root canals with apical periodontitis (Moore, 1987). Several factors contribute to the selection of micro-organisms. Primarily , the selection takes place among those micro-organisms entering the root canal, which depends on the pathway to the pulp. For example, a deep caries lesion may serve as a pathway and limit the number of possible microbial species. In addition,the host defense mechanisms in the infected but still vital pulp reduce the number of surviving species. Furthermore, environ-mental factors of the necrotic root canal, e.g., redox-potential and source of nutrients, give an advantage to species with pro-teolytic activity and ability to survive in anaerobic conditions.Finally, microbial interactions—either negative (such as compe-tition for nutrients, secreted toxic metabolites, and specific bacteriocins) or positive (i.e.,symbiosis of different spe-cies)—regulate the microflora of130Crit Rev Oral Biol Med14(2):128-137(2003)TABLE 2Micro-organisms Commonly Associated with Chronic Periodontitis, Apical Periodontitis, and Persistent Root Canal InfectionsDisease Group Micro-organismsReferencesChronic Gram-negative Fusobacterium nucleatum Moore and Moore, 1994periodontitisanaerobic rodsPorphyromonas gingivalis,Haffajee and Socransky, 1994Prevotella intermedia,Slots, 1999Campylobacter rectus,Hannula et al ., 2001Selenomonas spp.Treponema denticola Bacteroides forsythus Gram-negative Actinobacillusanaerobic rods actinomycetemcomitans Eikenella corrodens Gram-positive Peptostreptococcus micros anaerobic cocci Gram-positive Eubacterium spp.anaerobic rods Gram-positive Streptococcus intermedius facultative cocci YeastCandida albicans ApicalGram-negative Prevotella spp.Sundqvist, 1994periodontitisanaerobic rodsFusobacterium nucleatum,Sundqvist et al ., 1998Porphyromonas spp.Haapasalo, 1989Campylobacter rectus Selenomonas spp.Gram-positive Peptostreptococcus micros anaerobic cocci Gram-positive Eubacterium spp.anaerobic and Propionibacterium acnes facultative rods Actinomyces ctobacillus spp.Gram-positive Streptococcus spp.facultative cocciPersistent root Gram-positive Enterococcus faecalis Haapasalo et al ., 1983canal infectionfacultative cocci Streptococcus spp.Sirén et al ., 1997Waltimo et al ., 1997Gram-positive Peptostreptococcus spp.Sundqvist et al ., 1998anaerobic cocci Molander et al ., 1998Hancock et al ., 2001Gram-positive Actinomyces spp.anaerobic and facultative rods Gram-negative Bacteroides spp.anaerobic and facultative rods YeastCandida albicansthe infected root canal (Sundqvist, 1994).Apical periodontitis is a polymicrobial infection domina-ted by obligate anaerobes (Bergenholtz, 1974; Kanz and Henry, 1974; Sundqvist, 1976; Byström et al., 1985; Haapasalo, 1986; Sundqvist et al., 1989; Baumgartner and Falkler, 1991). Usually, the number of isolated species is between two and eight, and monoinfections are rare (K anz and Henry, 1974; Sundqvist, 1976, 1994; Haapasalo, 1986). Before root canal therapy, the most frequently isolated micro-organisms are: Gram-negative anaerobic rods, such as Prevotella spp., Porphyromonas spp., Fusobacterium nucleatum, Campylobacter rectus, and Selenomonas spp.; Gram-positive anaerobic cocci, such as Peptostreptococcus spp.; Gram-positive anaerobic and facultative rods, such as Eubacterium spp., Propionibacterium acnes, Actinomyces spp., and Lactobacillus spp.; and Gram-positive facultative Streptococcus species (Sundqvist, 1976; Haapasalo, 1986). The microbiology of root canal infections is still not clear in many regards, e.g., the data concerning the occurrence of uncultivable species such as spirochetes are scarce (Dahle et al., 1993).The literature on microbiological findings in persistent root canal infections is also relatively limited (Bender and Selzer, 1952; Grahnen and Krasse, 1963; Engström, 1964; Goldman and Pearson, 1969; Haapasalo et al., 1983; Ranta et al., 1988; Sirén et al., 1997; Molander et al., 1998). However, it is known that a few species are frequently isolated from persistent cases. These include the Enterococcus faecalis/faecium group, enteric Gram-negative facultative rods (i.e., coliforms), and Pseudomonas species (Engström, 1964; Haapasalo et al., 1983; Ranta et al., 1988; Molander et al., 1998; Sundqvist et al., 1998; Hancock et al., 2001; Love, 2001). Micro-organisms commonly associated with chronic periodontitis, apical periodontitis, and persistent root canal infections are listed in Table 2.Yeasts in Apical Periodontitis Microbiological investigations of apical periodontitis during the past 50 years have revealed that yeasts can be isolated from infected root canals (Grossman, 1952; Slack, 1953, 1957; Macdonald et al., 1957; Hobson, 1959; Goldman and Pearson, 1969; Matusow, 1981; Nair et al., 1990; Najzar-Fleger et al., 1992; Sen et al., 1995, 1997a,b; Waltimo et al., 1997; Molander et al., 1998). Slack (1953, 1957) reported that yeasts exist in about 5% of cases of apical periodontitis. According to Grossman (1952), as many as 17% of infected root canals may contain Candida species. Hobson (1959) reported that Candida albicans was often isolated from root canal infections, although their pathogenici-ty in the root canal was unclear. However, according to a case report, a pure culture of Candida albicans caused acute pulpal-alveolar cellulitis (Matusow, 1981).In another case report, C. albicans was found in root canals and in periapical granulomas of a patient suffering from chron-ic urticaria (Eidelmann et al., 1978). The complete cure of the patient was achieved only after the extraction of the infected teeth. Histological examination revealed that the granuloma exhibited an invasive Candida infection composed of acute and chronic granulation tissue along with hyphae and yeast cells. The root canal surfaces were covered by dense masses of yeast cells, and dentinal tubules were totally filled with hyphae. In addition to these cases, Damm et al. (1988) described two cases of cancer patients having dentinal candidosis. In the first case, carious dentin of the patient's deciduous teeth contained numerous oval to filamentous Candida cells. The teeth exhibit-ed either acute irreversible pulpitis or acute apical plete healing was accomplished after extraction of all deciduous teeth. The second case demonstrated exposed coro-nal dentin with heavy colonization by C. albicans. Pseudohyphae and yeast cells were present not only in pulp tissue but also in cervical and apical soft tissues. As seen in these cases, extensive invasion by fungi seems to be mostly associated with the immunocompromised state of the patients. However, Kinirons (1983) described a similar clinical case with no systemic illness.Nair et al. (1990) studied therapy-resistant root canal infec-tions and found micro-organisms in 6 of 9 specimens. Bacteria were shown in 4 of the 6 cases, while yeast-like organisms were found in 2 cases as judged by electron microscopy. The pres-ence of intraradicular fungi in the endodontically treated human teeth was associated with periapical lesions that per-sisted after treatment. Sen et al. (1995) observed bacteria and fungi with scanning electron microscopy in infected root canals and dentinal tubules associated with periapical lesions. They found that 4 out of 10 root canals were heavily infected with yeasts, confirming the association between yeasts and root canal infections. They formed dense but separate colonies, and, in one specimen, hyphal elements were also present. Since the patients in this study did not have any systemic disease, the presence of yeasts in root canals may be attributed to poor oral hygiene.In a report by Waltimo et al. (1997), the occurrence of yeasts was studied in 967 microbiological samples taken from cases of apical periodontitis not responding favorably to conventional treatment. Micro-organisms were found in 692 (72%) samples, whereas 275 (28%) showed no growth. Forty-eight fungal strains were isolated from 47 samples, which represented 7% of the culture-positive samples. The fungi were endomyceteous yeasts, and they were isolated either in pure culture (6 cases, 13%) or together with bacteria (41 cases, 87%). The identifica-tion of yeasts was carried out with conventional clinical labo-ratory procedures, showing results comparable with those of earlier studies. Almost all isolates belonged to the genus Candida, and C. albicans was the most common species. C.g labrata, C. g uilliermondii, C. inconspicua, and Geotrichum can-didum were also isolated.In studies of the initial microbial flora of root canal infec-tions, yeasts have usually not been found (Haapasalo, 1989; Sundqvist et al., 1989). However, according to a recent study of randomly selected patients with periapical radiolucencies, C. albicans was detected in 5 out of 24 samples (21%) taken from infected root canals by means of the polymerase chain-reaction-based (PCR) molecular detection technique (Baumgartner et al., 2000). The PCR was carried out conventionally with a detection limit of 10-4 ng of DNA. Although the material was limited, the high percentage may be due to the higher sensitivity of the method in comparison with detection of micro-organisms by conventional culture procedures. However, the finding indi-cates that yeasts may be present in low numbers at the start of root canal treatment, and that they may reach higher propor-tions during conventional treatment procedures. In another recent study, intact root canals with pulp necrosis were exam-ined microbiologically (Lana et al., 2001). C. tropicalis and S. cerevisiae were recovered from two canals (7.4%) before root canal therapy. C. guilliermondii and C. parapsilosis were cultivat-ed in the second and third collections, respectively, of root canal contents. According to these findings, it is also possible that yeasts which are common opportunistic pathogens of the oral14(2):128-137 (2003)Crit Rev Oral Biol Med131cavity gain access to the root canal as contaminants duringendodontic therapy (Sirén et al ., 1997). This emphasizes the importance of aseptic treatment procedures in the prevention of persistent infections. Regardless of the source and means of entry for yeasts into the root canal, their presence in cultivable numbers may have clinical importance in persistent cases.Influence of Necrotic Root Canalon Strain SelectionNecrotic root canals provide harsh ecological conditions for micro-organisms in comparison with other oral sites. The influ-ence of these conditions for yeast strain selection was examined in a recent study that compared the phenotypes and genotypes of C. albicans isolates from root canals and periodontal crevices (Waltimo et al ., 2001). Briefly , the phenotyping was based on the presence of 5 enzymes (valine arylamidase, phosphoamidase,alpha-glucosidase, beta-glucosidase, and N-acetyl-beta-glucosaminidase), ability of the strains to assimilate 11carbohydrates (glycerol, L-arabinose, xylose, adonitol,xylitol, sorbitol, methyl-D-glucoside, N-acetyl-D-glu-cosamine, sucrose, trehalose, and melezitose), and the resistance to boric acid (Williamson et al ., 1987).Genotyping was based on randomly amplified polymor-phic DNA (RAPD) profiles obtained with the use of two different primers.A total of 14 different phenotypes was found among the 37 root canal isolates of C. albicans . The majority of the isolates (26) were classifiable into three major phenotypes, described by Williamson et al . (1987):16 isolates (43.2%) belonged to phenotype A1R, 6(16.2%) to A1S, and 4 (10.8%) to B1S. Interestingly, C.albicans phenotype A1R, which was predominant, has been associated mainly with patients with symptomatic C. albicans infections but not with asymptomatic carri-ers. This may indicate a higher virulence of this pheno-type in comparison with other phenotypes (Xu and Samaranayake, 1995). The genotypic characterization with use of the combination of the two different primers yielded 32 different profiles for the 37 C. albicans strains,demonstrating high genotypic divergence of the iso-lates.Analysis of the current data implies genotypic het-erogeneity of C. albicans isolates from root canals in humans. However, frequently encountered phenotypes were similar to the ones reported from other oral and non-oral sources (Bostock et al ., 1993; Tsang et al ., 1995;Xu and Samaranayake, 1995; Matee et al ., 1996). This implies that phenotypically unusual strains of C. albicans are not frequently involved in root canal infections.Therefore, it seems that the root canal, an ecologically harsh niche with regard to redox-potential and nutrients supply, may not have an impact on strain selection that differs from those of other oral sites. Thus, it seems that a general characteristic of C. albicans is its ability to tolerate a wide variety of different environmental conditions.Accompanying Bacteria in Yeast InfectionsRecent studies have shown that yeasts can survive as a monoinfection of the root canal (Matusow, 1981; Waltimo et al ., 1997). However, they are usually found in mixed cultures together with bacteria. Yeasts may often be iso-lated together with facultative Gram-positive bacteria such as a- and non-hemolytic Streptococcus species, whereas Gram-negative isolates are rare (Waltimo et al ., 1997). The dom-inance of the facultative Gram-positive accompanying bacteria may be due to the special ecological conditions of the root canal during prolonged treatment, which could favor yeasts and streptococci. There may also be synergism between these micro-organisms. However, no or only negative association of Streptococcus spp. with other bacteria has been reported in root canals (Sundqvist, 1992). However, it has been reported that C.albicans may prolong the viability of -hemolytic streptococci (Burnet and Sherp, 1968). Furthermore, C. albicans co-aggre-gates with a variety of streptococci such as S. g ordonii, S.mutans, and S. sanguis (Holmes et al ., 1995; Nikawa et al ., 2001).This may promote their colonization and thus explain the con-comitant occurrence of these microbial species. Further investi-gations concerning microbial interactions are needed for a bet-132Crit Rev Oral Biol Med14(2):128-137(2003)Figure 1. Scanning electron micrograph of C. albicans blastospores on root canal surface in vitro . The bar indicates 10 m.Figure 2.Scanning electron micrograph of C. albicans hyphae penetrating a dentinal tubule. The bar indicates 2 m.。
不同程度镉污染对棉花生长和镉富集特征的影响
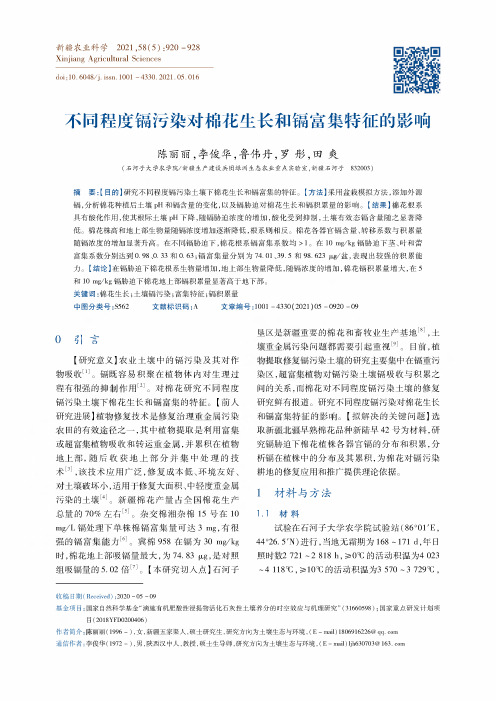
新疆农业科学 2021,53(5) :922 -923Xinjiang Agricultural Sciexcosdel : 14.0443/j ;算x. 1447 -4334. 9207.25.216不同程度镉污染对棉花生长和镉富集特征的影响陈丽丽,李俊华,鲁伟丹,罗彤,田 爽(石河子大学农学D/新疆生产建设6789生态农业;点实验?,新疆石河子334OO7)摘 要:【目的】开究不同程度镉污染土壤下棉花生长和镉富集的特征。
【方法】p 用盆栽模拟方法,添加外源镉,分析棉花种植后土壤pH 和镉含量的变化,以及镉胁迫对棉花生长和镉积累量的影响。
【结果】帛花根系 具有酸化作用,使其根际土壤pH 下降,随镉胁迫浓度的增加,酸化受到抑制,土壤有效态镉含量随之显著降低。
棉花株高和地上部生物量随镉浓度增加逐渐降低,根系则相反。
棉花各器官镉含量、转移系数与积累量随镉浓度的增加显著升高。
在不同镉胁迫下,棉花根系镉富集系数均>7。
在O mg/kg 镉胁迫下茎、叶和蕾 富集系数分别达到4. 98、4. 33和4. 63;镉富集量分别为酮.47、39. 5和93. 608 平盆,表现出较强的积累能力。
【结论】在镉胁迫下棉花根系生物量增加,地上部生物量降低,随镉浓度的增加,棉花镉积累量增大,在5和O mOkg 镉胁迫下棉花地上部镉积累量显著高于地下部。
关键词棉花生长;土壤镉污染;富集特征;镉积累量中图分类号:S562 文献标识码:A 文章编号:047 -4334(2421245 -0922 -094引言【研究意义】农业土壤中的镉污染及其对作物吸收[]。
镉既容易积聚在植物体内对生理过 程有很强的抑 用[2]。
对棉花研究不同程度镉污染土 棉花生长和镉 的 。
【前人 研究进展】植 复技术 复治理污染的有效之一,其中植物提取是利用或超 植物吸收和转运 ,并累积在植物地 ,随后收获地分 中处理的技术7],该技术应用广泛,修复成本低、环境友好、土壤破坏小,适用复大面积、中污染的土壤⑷。
根系分泌物的作用和调控功能
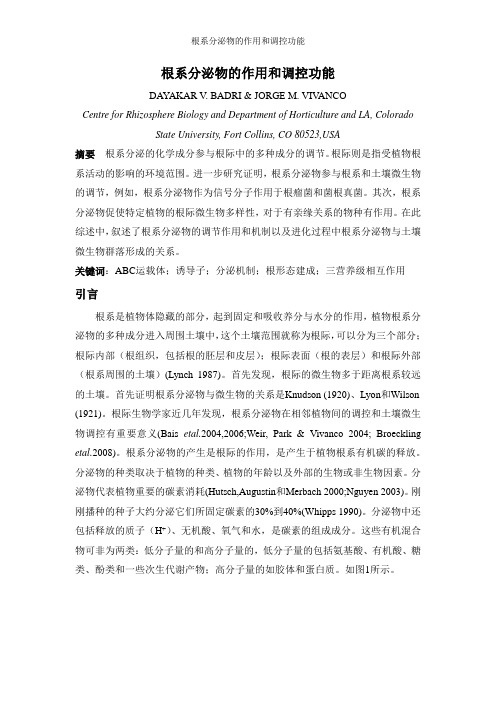
根系分泌物的作用和调控功能DAYAKAR V. BADRI & JORGE M. VIV ANCOCentre for Rhizosphere Biology and Department of Horticulture and LA, ColoradoState University, Fort Collins, CO 80523,USA摘要根系分泌的化学成分参与根际中的多种成分的调节。
根际则是指受植物根系活动的影响的环境范围。
进一步研究证明,根系分泌物参与根系和土壤微生物的调节,例如,根系分泌物作为信号分子作用于根瘤菌和菌根真菌。
其次,根系分泌物促使特定植物的根际微生物多样性,对于有亲缘关系的物种有作用。
在此综述中,叙述了根系分泌物的调节作用和机制以及进化过程中根系分泌物与土壤微生物群落形成的关系。
关键词:ABC运载体;诱导子;分泌机制;根形态建成;三营养级相互作用引言根系是植物体隐藏的部分,起到固定和吸收养分与水分的作用,植物根系分泌物的多种成分进入周围土壤中,这个土壤范围就称为根际,可以分为三个部分;根际内部(根组织,包括根的胚层和皮层);根际表面(根的表层)和根际外部(根系周围的土壤)(Lynch 1987)。
首先发现,根际的微生物多于距离根系较远的土壤。
首先证明根系分泌物与微生物的关系是Knudson (1920)、Lyon和Wilson (1921)。
根际生物学家近几年发现,根系分泌物在相邻植物间的调控和土壤微生物调控有重要意义(Bais etal.2004,2006;Weir, Park & Vivanco 2004; Broeckling etal.2008)。
根系分泌物的产生是根际的作用,是产生于植物根系有机碳的释放。
分泌物的种类取决于植物的种类、植物的年龄以及外部的生物或非生物因素。
分泌物代表植物重要的碳素消耗(Hutsch,Augustin和Merbach 2000;Nguyen 2003)。
2009-plant root growth, architecture and function - Hodge
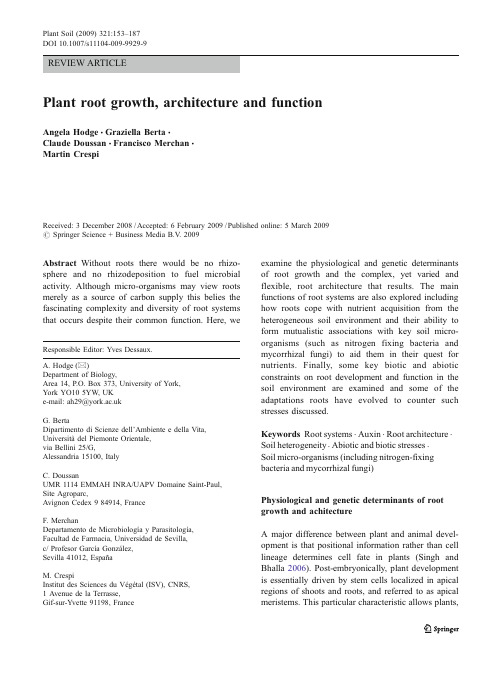
REVIEW ARTICLEPlant root growth,architecture and function Angela Hodge&Graziella Berta&Claude Doussan&Francisco Merchan&Martin CrespiReceived:3December2008/Accepted:6February2009/Published online:5March2009 #Springer Science+Business Media B.V.2009Abstract Without roots there would be no rhizo-sphere and no rhizodeposition to fuel microbial activity.Although micro-organisms may view roots merely as a source of carbon supply this belies the fascinating complexity and diversity of root systems that occurs despite their common function.Here,we examine the physiological and genetic determinants of root growth and the complex,yet varied and flexible,root architecture that results.The main functions of root systems are also explored including how roots cope with nutrient acquisition from the heterogeneous soil environment and their ability to form mutualistic associations with key soil micro-organisms(such as nitrogen fixing bacteria and mycorrhizal fungi)to aid them in their quest for nutrients.Finally,some key biotic and abiotic constraints on root development and function in the soil environment are examined and some of the adaptations roots have evolved to counter such stresses discussed.Keywords Root systems.Auxin.Root architecture. Soil heterogeneity.Abiotic and biotic stresses.Soil micro-organisms(including nitrogen-fixing bacteria and mycorrhizal fungi)Physiological and genetic determinants of root growth and achitectureA major difference between plant and animal devel-opment is that positional information rather than cell lineage determines cell fate in plants(Singh and Bhalla2006).Post-embryonically,plant development is essentially driven by stem cells localized in apical regions of shoots and roots,and referred to as apical meristems.This particular characteristic allows plants,Plant Soil(2009)321:153–187DOI10.1007/s11104-009-9929-9Responsible Editor:Yves Dessaux.A.Hodge(*)Department of Biology,Area14,P.O.Box373,University of York,York YO105YW,UKe-mail:ah29@G.BertaDipartimento di Scienze dell’Ambiente e della Vita, Universitàdel Piemonte Orientale,via Bellini25/G,Alessandria15100,ItalyC.DoussanUMR1114EMMAH INRA/UAPV Domaine Saint-Paul, Site Agroparc,Avignon Cedex984914,FranceF.MerchanDepartamento de Microbiología y Parasitología, Facultad de Farmacia,Universidad de Sevilla,c/Profesor García González,Sevilla41012,EspañaM.CrespiInstitut des Sciences du Végétal(ISV),CNRS,1Avenue de la Terrasse,Gif-sur-Yvette91198,Francewhich are sessile organisms,to adapt their morphol-ogy and organ development to the encountered environmental conditions.The spatial configuration of the root system(number and length of lateral organs),so-called root architecture,vary greatly depending on the plant species,soil composition, and particularly on water and mineral nutrients availability(Malamy2005).Plants can optimize their root architecture by initiating lateral root primordia and influencing growth of primary or lateral roots. The root system results from the coordinated control of both genetic endogenous programs(regulating growth and organogenesis)and the action of abiotic and biotic environmental stimuli(Malamy2005).The interactions between these extrinsic and intrinsic signals however complicate the dissection of specific transduction pathways.Such complex traits likely depending on multiple genes may be analyzed through quantitative genetics via the identification of quantitative trait loci(QTL)linked to root architecture (e.g.Mouchel et al.2004,Fitz Gerald et al.2006). Understanding the molecular mechanisms governing such developmental plasticity is therefore likely to be crucial for crop improvement in sustainable agricul-ture.In this section,we focus on hormonal and genetic determinants of root architecture.The embryonic root apical meristem(RAM) specification occurs very early in embryo develop-ment(Benfey and Scheres2000).The RAM con-stitutes the stem cell niche that eventually produces all below-ground organs,including lateral roots (Sabatini et al.2003).The cellular organization of Arabidopsis RAMs is very regular,with the initials of the various tissue types surrounding a quiescent center(QC).An asymmetric division of the hypoph-ysis generates a large basal daughter and a small apical daughter,called the lens-shaped cell,which is the progenitor of the QC.During root initiation,the QC appears to act like an organizing center that can direct surrounding cells to produce a set of initials (Aida et al.2004,Jenik et al.2007).Different mechanisms specify the initials above the QC that give rise to the ground tissue and the stele(proximal initials)as well as the initials below the QC that produce the root cap(distal initials).This root cap and the underlying root apical meristem form the root zone called the distal root apex,where cells divide actively.At certain distance from the RAM,the anticlinal and asymmetric divisions of a group of pericycle cells are the initial event of the formation of a lateral root.Several consequent divisions result in the formation of a dome-shape primordium that grows through outer cell layers and a meristem is estab-lished.Upon activation of this meristem,the lateral root emerges from the parental root and continue to grow in the soil(Malamy1995and references therein).Phytohormonal regulation of the root system:Auxin as a major playerThe different stages of root development are con-trolled and regulated by various phytohormones with auxin playing a major role(reviewed by Leyser 2006).In roots,auxin is involved in lateral root formation,maintenance of apical dominance and adventitious root formation.All these developmental events require correct auxin transport and signaling.During embryonic RAM formation many auxin-related mutants,such as those involved in its biosynthesis yuc1yuc4yuc10yuc11quadruple mutant (Cheng et al.2007),or mutants affected in transport or signaling(monopteros,bodenlos,auxin transport inhibitor resistant1(tir1)and related tir1/afb1–3 quadruple mutant(Dharmasiri et al.2005,Jenik et al. 2007)are unable to form the embryonic RAM.The auxin flux coming from the apical region of the embryo into the hypophysis leads to TIR1(and related redundant AFBs)pathway activation and induction of auxin-response genes such as PIN genes (coding for auxin efflux carriers),whose products will increase auxin transport and accumulation to further differentiate the hypothesis(Benkova et al.2003).Auxin also plays a major role in lateral root initiation and teral root development can be divided in different steps:primordium initia-tion and development,emergence,and meristem activation.Auxin local accumulation in Arabidopsis root pericycle cells adjacent to xylem vessels,triggers lateral root initiation by re-specifying these cells into lateral root founder cells(Dubrovsky et al.2008). Furthermore,it is also involved in the growth and organization of lateral root primordia and emergence from the parent root(Laskowski et al.2006).Indeed, mutants or transgenic lines with elevated auxin biosynthesis and endogenous levels of IAA display significant increased root branching(Seo et al.1998, King et al.1995).In addition,overexpression of theDFL1/GH3-6or the IAMT1genes,which encode enzymes modulating free IAA levels,results in a reduction in lateral root formation(Staswick et al. 2005;Qin et al.2005).Hence,normal auxin biosyn-thesis and homeostasis are necessary for lateral root initiation in Arabidopsis.However,overall auxin content is not always positively correlated with lateral root number(Ivanchenko et al.2006).Auxin transport into the regions where lateral root initiate also seems crucial for the regulation of root branching(Casimiro et al.2001).Functional analyses of mutants impaired in auxin transport,such as the aux1,axr4,and pin multiple mutants(Benkova et al. 2003;Marchant et al.2002),have demonstrated the crucial role of auxin transport in lateral root forma-tion.In the root,auxin is transported in both an acropetal(base-to-apex;Ljung et al.2005)and basipetal(apex-to-base)direction within inner and outer tissues of the root apex(Swarup and Bennett 2003).Studies using auxin transport inhibitors have shown that the polarity of auxin movement provides an important developmental signal during lateral root initiation(Casimiro et al.2001)and root gravitropism (Parry et al.2001).The strong bias in the direction of auxin transport within a tissue results from the asymmetrical cellular localization of an influx system involving the AUX1protein(Swarup and Bennett 2003)and an efflux apparatus with PIN-type efflux facilitators(reviewed by Paponov et al.2005).The auxin efflux system regulated by PIN protein family members is also crucial for lateral root development(Benkova et al.2003).Although not all pin mutations affect lateral root formation,multiple pin mutants have dramatic defects in root patterning, including lateral root development(Benkova et al. 2003;Blilou et al.2005).Cellular localisation of PIN proteins is also important as a gnom mutant allele is defective in PIN-dependent developmental processes, including lateral root primordium development,pre-sumably due to disorganized PIN1localization (Geldner et al.2004).When auxin reaches the target tissue it induces a transcriptional response.Several auxin-responsive genes and gene families involved in auxin signalling have been identified,of which the Aux/IAAs and ARFs are the best studied.Auxin induces expression of Aux/IAA proteins,which in many cases reduces the sensitivity of cells toward auxin.This induction is mediated by ARF proteins that bind to the auxin-responsive elements(AuxREs)in the promoters of auxin-responsive genes,and activate or repress transcription through interaction with specific Aux/ IAA proteins(Liscum and Reed2002).These loops represent extensive feedback as well as feed-forward regulation(for further information on Aux/IAA pro-teins,their degradation and their interaction with ARF transcription factors see the review by Benjamins and Scheres2008).Alterations in these control systems affect several auxin-dependent plant pathways includ-ing root development.For example,normal differen-tiation of a root cap from the distal initials depends on a pair of redundantly acting auxin response factors, ARF10and ARF16(Wang et al.2005).In double mutants,the root cap initials overproliferate,thereby generating a mass of undifferentiated progeny. Another example are the gain-of-function solitary root(slr)mutants which carries a stabilizing mutation in the IAA14negative regulator of auxin signaling and cannot form lateral root primordia.Detailed analyses of the slr mutant demonstrated that the slr mutation blocks early cell divisions during initiation (Fukaki et al.2002)Other hormones involved in the control of root developmentAlthough auxin plays a fundamental role in root growth and development,several other phytorho-mones modulate auxin action and consequently affect root development and architecture.In contrast to auxin,exogenous cytokinin application suppresses lateral root formation,and transgenic Arabidopsis plants with decreased cytokinin levels display in-creased root branching and enhanced primary root growth(Werner et al.2003).The notion that cytokinin negatively regulates root growth has also been verified by studies of cytokinin perception and signalling.For instance,double mutants for the redundant Arabidopsis cytokinin receptors AHK2 and AHK3display a faster growing primary root and greatly increased root branching(Riefler et al. 2006).To identify the stage of lateral root develop-ment sensitive to cytokinin,Laplaze et al.(2007) performed transactivation experiments that revealed that xylem-pole pericycle cells,but not lateral root primordial,are sensitive to cytokinin.Cytokinins perturb the expression of PIN genes in lateral root founder cells and prevent the formation of the auxingradient required to pattern the primordia.Recently cytokinin have also been shown to induce differenti-ation of cells situated between the root meristem and the elongation zone(Dello Ioio et al.2007),providing new insights on the molecular mechanisms involved in root meristem maintenance.The actively growing primary root of dicotyledon plants may exhibit apical dominance forcing their lateral roots to develop further away from the root tip (Lloret and Casero2002).Root apical dominance may be triggered by the root-cap-synthesized cytoki-nin and the balance between auxin and cytokinin regulates root gravitropism,a major determinant of root architecture(Aloni et al.2006).The control of lateral root initiation depends on the primary root vascular system which conveys spatial information as well as photosynthetic carbon to the newly formed primordial(Parizot et al.2008).Three types of vascular differentiation can be distinguished:(1) primary differentiation,which occurs in cells origi-nating from the primary vascular meristem,the procambium;(2)secondary differentiation,where the derivates originate from the vascular cambium; and(3)regenerative differentiation,in which the vascular elements re-differentiate from parenchyma cells at lateral root junctions.The vascular tissues are induced by polar auxin movement along the plant body,from the hydathodes of young leaves downward to the root tips.Cytokinin by itself does not induce vascular tissues,but in the presence of auxin,it promotes vascular differentiation and regeneration,a critical process for the establishment of a root system (Aloni et al.2006).Another hormone involved in lateral root for-mation is ethylene.Treatments that induce C2H4 production(i.e.flooding)promote adventitious and lateral root formation(Mergemann and Sauter2000). Ethylene stimulates auxin biosynthesis and basipetal auxin transport toward the elongation zone,where it activates a local auxin response leading to inhibition of cell elongation.Stepanova et al.(2005)showed that ethylene-triggered inhibition of root growth is mediated by the action of the weak ethylene insensitive2/anthranilate synthaseα1(WEI2/ASA1) and wei7/anthranilate synthaseß1(ASB1)genes that encode rate-limiting tryptophan biosynthesis enzymes In addition,ethylene modulates the tran-scription of several components of the auxin trans-port machinery and induces a local activation of the auxin signaling pathway to regulate root growth (Ruzicka et al.2007).Abscisic acid(ABA),a universal stress hor-mone,plays a significant role in stimulating elongation of the main root and emergence of lateral roots in response to drought(De Smet et al. 2006b).As with other hormones,the complexity of the plant responses induced by this hormone makes it difficult to distinguish primary from secondary effects(e.g.stomata closure affects water movement in the plant).In general,ABA has an antagonistic effect on lateral root primordial formation and emergence to auxin,which initiates lateral roots (Malamy2005).This role of ABA in the control of the activity of the lateral root meristems has a significant impact on the final size and architecture of the root system(De Smet et al.2006b).Indeed, responsiveness to certain abiotic factors is lost in certain ABA signaling mutants(Signora et al.2001; He et al.2005;Fujii et al.2007)such as the systemic inhibition of lateral root formation in high N conditions in the ABA-insensitive mutant abi4 (Signora et al.2001).Genetic and genomic approaches to analyze root growth and architectureForward and reverse genetic approaches have been undertaken to elucidate the genetic control of lateral root formation.As expected,most of the lateral root mutants(approximately40%)identified in A.thaliana are affected in a specific component of the auxin pathways.Their phenotypes can usually be rescued or mimicked through auxin application.Several mutants having lateral root phenotypes(De Smet et al.2006a) have been linked to auxin signaling(axr mutants,tir1, msg2-1,shy2),auxin transport(aux1and pin mutants),and auxin homeostasis(alf1,ydk1,dfl1). Analysis of these mutants has led to the identification of several genes that regulate lateral root formation and/or coordinate this process in response to environ-mental cues(reviewed in Osmont et al.2007).Few Arabidopsis mutants such as the previously men-tioned slr-1/AUXIAA14(Fukaki et al.2002)and alf4-1(Celenza et al.1995),are not capable of initiating any lateral roots.The encoded ALF4protein is conserved among plants and has no similarities to proteins from other kingdoms.The Arabidopsis gene is involved in lateral root initiation but not in primaryroot formation as the alf4-1mutant forms a primary root.DiDonato et al.(2004)have shown that ALF4 functions are required to maintain the pericycle in a mitosis-competent state needed for lateral root forma-tion.Other mutants are affected in vascular tissues and consequently cannot produce lateral roots(e.g. Ohashi-Ito and Bergmann2007).In contrast,ectopic expression of PLT genes, coding for AP2-type transcription factors,trigger de novo formation of roots from shoot structures.PLT1 was isolated by reverse genetics technologies based on the identification of a QC–and stem-cell–specific enhancer trap line.Indeed,PLT genes are pivotal determinants of QC and stem cell identity(Aida et al.2004).Although plt1mutants only display mild root growth defects,the establishment and mainte-nance of the QC and stem cells in the root meristem, are severely impaired in the plt1plt2double mutant. NAC1is another transcription factor implicated in lateral root development since its overexpression enhances lateral root initiation(Xie et al.2002).A genetic framework for the control of cell division and differentiation in root meristematic cells have been recently proposed(Dello Ioio et al.2008).A second strategy used to identify non-essential modulators of root development,is exploiting natural genetic variation by analysis of quantitative trait loci(QTL).QTL mapping has the advantage of identifying genomic regions containing genes with subtle effects masked in a particular genetic background.For instance,in A.thaliana and maize QTLs linked to root architecture have been identified (Mouchel et al.2004;Tuberosa et al.2002;Loudet et al.2005).Exploring the polymorphisms underlying natural variation can identify the DNA sequence changes that lead to a modified root system architec-ture in nature.In recent years,several new genomics resources and tools(e.g.genome sequences,tens of thousands of molecular markers,microarrays,and knock-out collections)have become available to assist in QTL mapping and cloning,even if these genes have subtle effects on phenotype(see Paran and Zamir2003).For example,quantitative morpholog-ical and physiological variation analysis of a cross between isogenized Arabidopsis accessions revealed that the BREVIS RADIX(BRX)transcription factor controls the extent of cell proliferation and elonga-tion in the growth zone of the root tip(Mouchel et al. 2004).Genomics have provided new tools to explore downstream and upstream genes of broad regulatory signals such as hormone-responsive factors.Tran-scriptional changes during root branching in Arabi-dopsis have been monitored to identify key regulators of root architecture(Himanen et al.2004),including several core cell cycle genes.In addition,Vanneste et al.(2005)compared the early transcriptome of lateral root formation using synchronised induction of these organs in wild type and slr mutants.This revealed negative and positive feedback mechanisms that regulate auxin homeostasis and signalling in the pericyle initial cells.The advent of genomic resources have allowed the combination of global profiling with novel biological approaches such as enhancer trap lines driving green fluorescent protein(GFP)expression in specific root cell types(Brady et al.2007).These authors used transgenic lines that expressed GFP in specific root tissues to isolate corresponding fluores-cent protoplast populations derived from them and characterise their expression patterns using micro-arrays.Thus were able to determine which genes were expressed and at what level in a particular root cell type.Recently,these transgenic plants were used to characterise the transcriptional response to high salinity of different cell layers and developmental stages of the Arabidopsis root(Dinneny et al.2008). Transcriptional responses are highly constrained by developmental parameters further revealing interac-tions between developmental fates and environmental stresses.Genomic approaches mainly rely on the expression patterns of protein-coding genes.However,the dis-covery of small RNAs(microRNAs,miRNA;and small interfering RNAs,siRNA)in the last decade altered the paradigm that protein coding genes are the only significant components of gene regulatory net-works(Chapman and Carrington2007).Small RNAs are involved in a variety of phenomena that are essential for genome stability,establishment or main-tenance of organ identity,adaptive responses to biotic and abiotic stresses.In plants,MIRNAs are genes generally encoded in intergenic regions whose matu-ration requires a particular type III RNase named DICER-LIKE1(DCL1)(Chapman and Carrington 2007).The small mature miRNA is then incorporated in a protein complex,the so-called RISC(RNA-Induced Silencing Complex)that can recognizemRNAs partially complementary to the miRNA nucleotide sequence.This recognition event mediated by the RISC-loaded miRNA leads to cleavage or translational inhibition of the target mRNA.Mutants affected in miRNA metabolism(biosynthesis,action and transport as dcl1,ago1,hen1,hyl1,hst1,se)show pleiotropic phenotypes confirming the role of miR-NAs in diverse developmental processes(Chapman and Carrington2007).Several MIRNAs have been involved in root development.For example,Arabidopsis mir164a and mir164b mutant plants produced more lateral roots than wild-type plants(Guo et al.2005).These phenotypes are similar to those obtained by NAC1 overexpression,a transcription factor targetted by miR164(Xie et al.2002).Overexpression of miRNA-resistant NAC1mRNA results in slight increases in lateral root numbers.In addition,the auxin-related transcription factors ARF10and ARF16 are also targets of three related microRNA(miRNA) genes,miR160a,miR160b and miR160c.These miR-NAs limit the expression domain of ARF10and ARF16 to the columella,and have a complementary pattern. The over-expression of a miRNA-resistant ARF16 mRNA under its own promoter results in pleiotropic developmental defects.MIR160overexpressing plants, in which the expression of ARF10and ARF16is repressed,and the arf10-2arf16-2double mutants display the same root tip defect,with uncontrolled cell division and blocked cell differentiation in the root distal region showing a tumor-like root apex and loss of gravity-sensing(Wang et al.2005).Apart from these examples,due to the large diversity of these novel regulatory RNAs,it is likely that many other miRNAs or other small RNAs participate in the regulation of root development and architecture.Root meristematic cells integrate signals from the environment to regulate specific developmental responses and cope with external constraints.This post-embryonic growth and development requires the activation of hormone homeostasis and signalling pathways,transcriptional regulation by specific tran-scription factors and post-transcriptional regulation of developmental regulators by non-coding RNAs. These regulatory mechanisms may be particularly relevant to adjust differentiation processes to the environmental conditions encountered during growth, notably on primary and lateral root developmental programs.Root system achitectureRoot systems and architecture definitionsSoil is a complex medium with high spatial and temporal environmental variability at a wide range of scales,including those relevant to plant roots.The root system,with its extensive but structured devel-opment,can be considered as an evolutionary response to such spatio-temporal variability in re-source supply and associated constraints upon growth (Harper et al.1991).As a consequence,the extension in space and time of the root system is governed by genetically driven developmental rules which are modulated by environmental conditions.As demon-strated by QTL mapping which recently revealed that root morphology is in most cases regulated by a suite of small-effect loci that interact with the environment (de Dorlodot et al.2007).The architecture of a root system is the result of developmental processes and is a dynamic notion. Root architecture addresses two important concepts: the shape of the root system and its structure.The shape defines the location of roots in space and the way the root system occupies the soil.Its quantifica-tion is generally achieved by measuring variables such as root depth,lateral root expansion and root length densities.In contrast,root structure describes the variety of the components constituting the root system(roots and root segments)and their relation-ship(e.g.topology:connexion between roots;root gradients).While,root differentiation has important impacts upon structure—function relations(Clarkson 1996).The rhizosphere(i.e.the volume of soil around living plant roots that is influenced by root activity; Hinsinger et al.2005),is often simply thought of as a cylindrical shape around the root.However,this oversimplification does not account for integration at the root system level or for the inherent complexity of root systems which arise from geometry,temporal dynamics and the heterogeneous aspects of roots. These complexities are incorporated into the concept of root architecture.Root geometry is complex because of the specific motion in space of each root, the relative locations between roots and the possible overlapping of their zones of influence.The temporal dynamic comes both from the growth of the different root axes and from physiological processes associatedwith root segments (i.e.tissue differentiation)result-ing in temporal and spatial variability of function along the root axes.The diversity among roots within the root system and soil heterogeneity further increase this variability.Root classification and elaboration of the root system It has long been recognized that the in situ morphol-ogy of a root system can be complex and may vary greatly,even within a species (Weaver 1926;Cannon 1949;Kutschera 1960),reflecting the interplay be-tween developmental processes and environmental constraints (Fig.1).Consequently,the complexity of root systems has led to a number of different classification systems.These classifications can be based on:branch structure (topology)(Fitter 1987;2002),root activity (Wahid 2000)or development (Cannon 1949).The latter is the more classical approach and is useful in understanding growth as well as obtaining a more global view of root architecture,for example,in relation to plant habitat.Thus,developmental classification has been widely used in modelling approaches to simulate architecture (cf section below).From a developmental stand point roots are classified according to their ontogenesis into three main categories:primary,nodal and lateral roots(Cannon 1949;Harper et al.1991;Klepper 1992),the formation of which has been shown to be genetically separable (Hochholdinger et al.2004).This classification also reflects differences between monocot –and dicotyledon species:dicots root system is derived from primary roots and lateral branching (primary root system),with roots that may exhibit radial growth.Depending on the extension of laterals relative to the primary axis,the morphology of the root system will vary between taprooted and diffuse or fasciculate (Fig.1).In monocots,root systems derive not only from branching of primary roots but also from emmited nodal roots (adventitious root system).Monocot roots do not undergo secondary radial growth.The primary root differentiates from the seed ’s radicle already present in the seed embryo.This generally gives rise to a single-axis root system,or taproot system,with dominant vertical growth (gravitropism).Adventitious (or nodal)roots differ-entiate from organs other than roots (e.g.rhizomes,stems etc)and are initiated at precise locations (near stem nodes for example)with a defined temporal pattern,in coordination with the development of the shoot.They are often abundant and give rise to a fasciculated root system.Adventitious roots are much less sensitive to gravitropism than primary roots (Klepper 1992).Lateral roots originate from the branching of a parent axis,often at near rightangles,Fig.1Diversity of root systems.On the top row,root systems are little branched,while more profusely branched on the bottom row.Dominance of a single main axis increases from left to right (from Kutschera 1960)。
缺氧诱导因子_1与干细胞功能调控
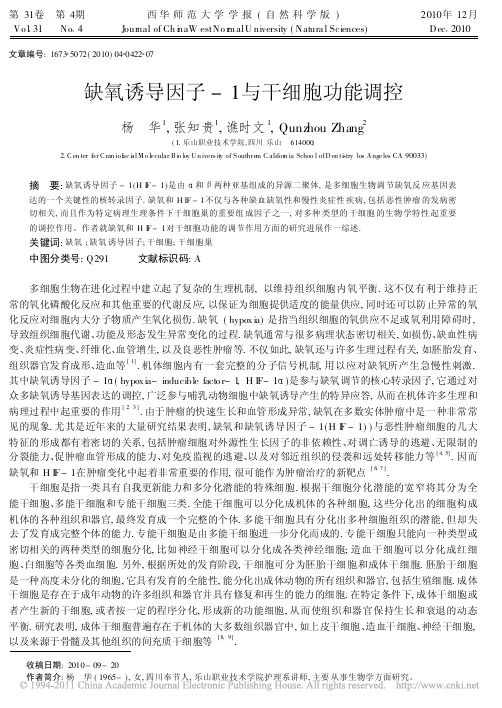
, 通过 H IF - 1
及其他相关的信号途径对干细胞的生物学特性起着重要的调控作用. 此文拟重点就低氧及低氧诱导因子对 干细胞调控的研究进展等作一综述.
1 H IF- 1 蛋白的结构特点
人的 H IF - 1 基因定位于人染色体 14Q21- Q24 , 其蛋白质表达产物为含 及 亚基的异源二聚体. 其 中 O2主要通过 亚基对 H IF - 1 的活性进行调节, 而 亚基是 H IF - 1的结构性亚基, 其在细胞内的表达不 受氧浓度变化的影响 . 及 亚基在 N 末端都有 一个碱性螺旋 - 环 - 螺旋结构域 ( basic he lix- loophe lix ; b H LH ) ; 紧 随其后是 一个保 守的 PAS ( per arnt si m ) 区. b H LH 和 PAS 域的功能主要和两个亚基的聚 合及靶基因特定 DNA 序列的识别有关 . 亚基为多种转录因子所共有, 又称芳烃受体核转运蛋白 ( ARNT ) , 由 789个氨基酸组成 , 分子 量为 94kDa , 只有一 个定位于 C - 末 端的转录 激活区 ( transact iv ation dom ain , TAD) . H IF - 1 的 亚基由 826 个氨基酸组成, 分子量为 120kDa , 其 C 末端含有两个转录激活结构域 , 即 N 末端 TAD 和 C 末端 TAD, 而和 N 末端 TAD 域相重叠并富含丝氨酸、 亮氨酸和苏氨酸的结构域, 叫做氧依赖 降解区 ( oxygen- dependen t deg rada tio n dom ain , ODD) . TAD 域主要通过与 RNA 聚合酶相互作用 , 促进 H IF1 的转录活化, 而 ODD 域则主要与氧诱导的 H IF- 1 降解有关. 另外在 亚基的 N 末端, 以及 N 末端 TAD 和 C 末端 TAD 之间还分别有一个特殊的结构域 , 叫做核定位信号域 ( nuclear lo ca lizatio n sig na, l NLS). 它主要与 H IF - 1由胞浆向胞核内迁移有关. 虽然 亚基在胞内不受氧浓度调节, 但 亚基需与 亚基形成异二聚体才能 [2 , 3] 转移到细胞核内发挥其转录活性. H I F - 2 和 H IF - 3 的结构及生物学特性与 H IF- 1 相似 .
鱼腥草素钠对金黄色葡萄球菌自溶抑制的

鱼腥草素钠对金黄色葡萄球菌自溶抑制的转录组研究与功能性分析刘丽慧1,梁俊超1,李扬1,郭娜2,申凤鸽1,邢明勋1,王学林1,吴秀萍1,于录1(1. 吉林大学人兽共患病研究所,人兽共患病研究教育部重点实验室,吉林大学畜牧兽医学院,长春130062;2. 吉林大学食品质量与安全系,长春130062)摘要:本实验中,鱼腥草素钠对21 株金黄葡萄球菌具有体外抗菌活性。
鱼腥草素钠可降低Triton X-100 对金黄色葡萄球菌自溶的诱导作用,而酶谱电泳分析和自溶酶定量检测方法证实鱼腥草素钠可降低金黄色葡萄球菌胞外的胞壁质水解酶的活性。
芯片结果显示经鱼腥草素钠作用后的菌株与对照菌株相比,自溶素基因atl, sle1, cidA 和lytN 转录的抑制水平与自溶抑制因子基因lrgAB 和sarA 的诱导水平一致且自溶正调控因子基因agrA 和RNAIII 下调。
负调控因子基因lytSR, mgrA 和arlS 表达水平下降并不能说明自溶抑制是因鱼腥草素钠作用所致。
而大量毒力因子基因转录抑制表明鱼腥草素钠是通过一条共享作用途径影响自溶和毒力因子基因的。
除此之外,一些重要基因的转录也受到鱼腥草素钠的影响。
芯片中的部分结果我们已通过RT-PCR 验证。
关键词:金黄色葡萄球菌;鱼腥草素钠;基因芯片;转录中图分类号:S432.4+2 文献标识码:AIn vitro activity of isoimperatorin in combination withrifampicin, Transcriptional Profiling and Functional Analysis Reveals Sodium Houttuyfonate-mediated Inhibition of Autolysis in Staphylococcus aureus Liu Lihui1, Liang Junchao1, LiYang1, Guo Na2, Shen Fengge1, Xing Mingxun1,Wang Xuelin1, Wu Xiuping1, Yu Lu1(1. Key Laboratory of Zoonosis Research, Ministry of Education, Institute of Zoonosis, College ofAnimal Science and Veterinary Medicine, Jilin University, ChangChun 130062;2. Department of Food Quality and Safty, Jilin University, ChangChun 130062)Abstract: Sodium houttuyfonate (SH) showed in vitro antibacterial activity against 21 Staphylococcus aureus (S. aureus) strains. Triton X-100-induced autolysis was significantly decreased by SH in S. aureus ATCC 25923, and zymographic analysis and quantitative bacteriolytic assays demonstratedSH-mediated reduction of extracellular murein hydrolase activity in these cells. Microarray results showed decreased levels of autolysin atl, sle1, cidA and lytN transcripts in the SH-treated strain as compared to the control strain that were consistent with the induction of the autolytic repressors lrgAB and sarA and with the downregulation of the positive regulators agrA and RNAIII. The reduced expression of the additional negative regulators lytSR, mgrA, and arlS fails to explain the inhibition of autolysi s triggered by SH. In addition, the transcription of a large number of virulence factors were inhibited, indicating that SH affects autolysis and virulence genes via a shared pathway. Moreover, the transcription of several important genes such as those of the iron-regulated surface determinant system was also affected by SH. Some of the microarray results were confirmed by real-time RT-PCR. Keywords:Staphylococcus aureus; sodium houttuyfonate; GeneChip; transcription基金项目:国家自然基金(30871889);教育部新教师基金(200801831051);国家科技重大专项子课题(2008ZX10301);吉林省科技发展计划项目(20080565);吉林大学基础科研业务费;吉林大学农学部人才建设基金作者简介:刘丽慧,(1987-),女,硕士通信联系人:于录,(1970-),男,教授,.E-mail:***************联用或糖肽类万古霉素[6]和替考拉宁[7]联用可降低细菌自溶。
朱章志运用扶正祛邪法论治糖尿病经验
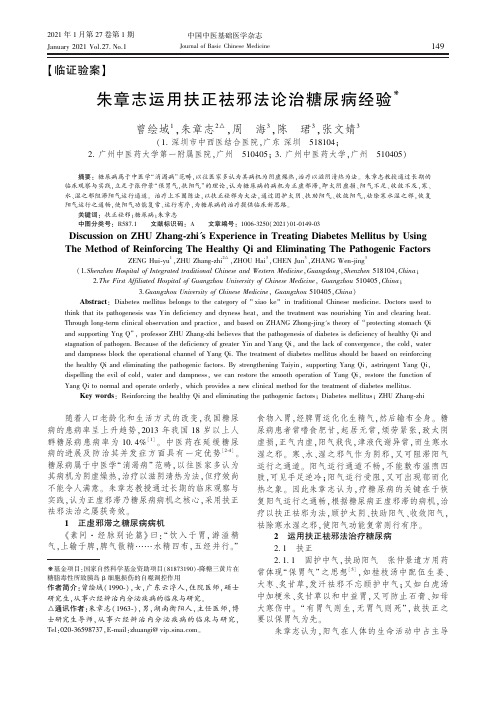
ʌ临证验案ɔ朱章志运用扶正祛邪法论治糖尿病经验❋曾绘域1,朱章志2ә,周㊀海3,陈㊀珺3,张文婧3(1.深圳市中西医结合医院,广东深圳㊀518104;2.广州中医药大学第一附属医院,广州㊀510405;3.广州中医药大学,广州㊀510405)㊀㊀摘要:糖尿病属于中医学 消渴病 范畴,以往医家多认为其病机为阴虚燥热,治疗以滋阴清热为法㊂朱章志教授通过长期的临床观察与实践,立足于张仲景 保胃气,扶阳气 的理论,认为糖尿病的病机为正虚邪滞,即太阴虚损㊁阳气不足㊁收敛不及,寒㊁水㊁湿之邪阻滞阳气运行通道㊂治疗上不囿陈法,以扶正祛邪为大法,通过固护太阴㊁扶助阳气㊁收敛阳气,祛除寒水湿之邪,恢复阳气运行之通畅,使阳气功能复常㊁运行有序,为糖尿病的治疗提供临床新思路㊂㊀㊀关键词:扶正祛邪;糖尿病;朱章志㊀㊀中图分类号:R587.1㊀㊀文献标识码:A㊀㊀文章编号:1006-3250(2021)01-0149-03Discussion on ZHU Zhang-zhi's Experience in Treating Diabetes Mellitus by Using The Method of Reinforcing The Healthy Qi and Eliminating The Pathogenic FactorsZENG Hui-yu 1,ZHU Zhang-zhi 2ә,ZHOU Hai 3,CHEN Jun 3,ZHANG Wen-jing 3(1.Shenzhen Hospital of Integrated traditional Chinese and Western Medicine,Guangdong,Shenzhen 518104,China;2.The First Affiliated Hospital of Guangzhou University of Chinese Medicine,Guangzhou 510405,China;3.Guangzhou University of Chinese Medicine,Guangzhou 510405,China)㊀㊀Abstract :Diabetes mellitus belongs to the category of "xiao ke"in traditional Chinese medicine.Doctors used to think that its pathogenesis was Yin deficiency and dryness heat ,and the treatment was nourishing Yin and clearing heat.Through long-term clinical observation and practice ,and based on ZHANG Zhong-jing's theory of protecting stomach Qi and supporting Yng Q ,professor ZHU Zhang-zhi believes that the pathogenesis of diabetes is deficiency of healthy Qi and stagnation of pathogen.Because of the deficiency of greater Yin and Yang Qi ,and the lack of convergence ,the cold ,water and dampness block the operational channel of Yang Qi.The treatment of diabetes mellitus should be based on reinforcing the healthy Qi and eliminating the pathogenic factors.By strengthening Taiyin ,supporting Yang Qi ,astringent Yang Qi ,dispelling the evil of cold ,water and dampness ,we can restore the smooth operation of Yang Qi ,restore the function of Yang Qi to normal and operate orderly ,which provides a new clinical method for the treatment of diabetes mellitus.㊀㊀Key words :Reinforcing the healthy Qi and eliminating the pathogenic factors ;Diabetes mellitus ;ZHU Zhang-zhi❋基金项目:国家自然科学基金资助项目(81873190)-降糖三黄片在糖脂毒性所致胰岛β细胞损伤的自噬调控作用作者简介:曾绘域(1990-),女,广东云浮人,住院医师,硕士研究生,从事六经辨治内分泌疾病的临床与研究㊂ә通讯作者:朱章志(1963-),男,湖南衡阳人,主任医师,博士研究生导师,从事六经辨治内分泌疾病的临床与研究,Tel :************,E-mail :zhuangi@ ㊂㊀㊀随着人口老龄化和生活方式的改变,我国糖尿病的患病率呈上升趋势,2013年我国18岁以上人群糖尿病患病率为10.4%[1]㊂中医药在延缓糖尿病的进展及防治其并发症方面具有一定优势[2-4]㊂糖尿病属于中医学 消渴病 范畴,以往医家多认为其病机为阴虚燥热,治疗以滋阴清热为法,但疗效尚不能令人满意㊂朱章志教授通过长期的临床观察与实践,认为正虚邪滞乃糖尿病病机之核心,采用扶正祛邪法治之屡获奇效㊂1㊀正虚邪滞之糖尿病病机‘素问㊃经脉别论篇“曰: 饮入于胃,游溢精气,上输于脾,脾气散精 水精四布,五经并行㊂食物入胃,经脾胃运化化生精气,然后输布全身㊂糖尿病患者常嗜食肥甘,起居无常,烦劳紧张,致太阴虚损,正气内虚,阳气戕伐,津液代谢异常,而生寒水湿之邪㊂寒㊁水㊁湿之邪气作为阴邪,又可阻滞阳气运行之通道㊂阳气运行通道不畅,不能敷布温煦四肢,可见手足逆冷;阳气运行受阻,又可出现郁而化热之象㊂因此朱章志认为,疗糖尿病的关键在于恢复阳气运行之通畅,根据糖尿病正虚邪滞的病机,治疗以扶正祛邪为法,顾护太阴㊁扶助阳气㊁收敛阳气,祛除寒水湿之邪,使阳气功能复常则行有序㊂2㊀运用扶正祛邪法治疗糖尿病2.1㊀扶正2.1.1㊀固护中气,扶助阳气㊀张仲景遣方用药常体现 保胃气 之思想[5],如桂枝汤中配伍生姜㊁大枣㊁炙甘草,发汗祛邪不忘顾护中气;又如白虎汤中加梗米㊁炙甘草以和中益胃,又可防止石膏㊁知母大寒伤中㊂ 有胃气则生,无胃气则死 ,故扶正之要以保胃气为先㊂朱章志认为,阳气在人体的生命活动中占主导9412021年1月第27卷第1期January 2021Vol.27.No.1㊀㊀㊀㊀㊀㊀中国中医基础医学杂志Journal of Basic Chinese Medicine地位㊂‘素问㊃生气通天论篇“曰: 阳气者若天与日,失其所则折寿而不彰 是故阳因而上,卫外者也㊂ ‘黄帝内经“把阳气比作太阳,阳气运行失常可致短寿㊂阳气具有抵御外邪㊁护卫生命㊁维持机体生命活动的作用,津液的气化㊁血液的运行均需阳气的温煦与推动㊂因此,在人体的阴阳平衡中阳气起着主导作用㊂朱章志认为,正气虚衰㊁太阴虚损㊁阳气不足是糖尿病发生发展之根本原因,因此扶正首当 固护中气,扶助阳气 ,故常以附子理中汤为底方,固护中宫㊂太阴脾土居中央,犹如足球比赛之中场,能联系前锋与后卫进可攻退可守,进可充养肺卫之气抵御外邪,退可顾护少阴以防寒邪内陷㊂‘四圣心源㊃卷二太阴湿土“提到: 湿者,太阴土气之所化也故胃家之燥,不敌脾家之湿,病则土燥者少而土湿者多也㊂[6] 阴脾土易挟寒湿,附子理中汤功善固护中气㊁温补脾阳而散寒湿,为治疗太阴阳虚寒湿之要方㊂方中附子辛温大热,补坎中真阳,又能散寒湿,荡去群阴;干姜去脏腑沉寒痼冷,温暖脾土,复兴火种;人参被誉为 百草之王 能大补元气,为扶正固本之极品;白术味苦性温,功善健脾燥湿,乃扶植太阴之要药;炙甘草善益气补中,调和药性,诸药合用以收培补中阳㊁散寒除湿之效㊂若其人神疲懒言,气虚较甚,在附子理中汤的基础上可重用红参㊁北芪以大补元气,健脾益气;若其人四肢不温㊁肢体困重㊁寒湿较重者,可加重附子㊁干姜之量,并加细辛㊁吴茱萸以散久寒;若其人口干口苦㊁舌苔黄腻㊁大便黏滞不爽兼夹湿热之象,可仿当归拈痛汤之意,加茵陈㊁当归㊁黄芩以利湿清热㊂2.1.2㊀收敛阳气,阳密乃固㊀朱章志认为, 阴 可理解为 阳气 的收敛㊁收藏状态,糖尿病 阴虚燥热 之象乃阳气不足㊁收敛不及㊁升发太过所致[7]㊂‘素问㊃生气通天论篇“提到: 阳气者,烦劳则张 ㊂现代人起居无节,以妄为常,阳气因而不能潜藏,常常浮越于外容易出现假热之象,医者不察,妄投清热泻火之品,实乃雪上加霜㊂ 凡阴阳之要,阳密乃固 ,收敛阳气即是扶正,犹如太极之能收能放,收敛是为了聚集能量,阳气固密,正气才能强盛,方能更好的制敌㊂朱章志常用砂仁㊁肉桂㊁白芍㊁山萸肉㊁泽泻等药物收敛阳气㊂砂仁辛温,既能宣太阴之寒湿,又能纳气归肾,使阳气收敛于少阴,少火生气㊂‘本草经疏“提到: 缩砂蜜,辛能散,又能润 辛以润肾,故使气下行 气下则气得归元㊂[8] 肉桂引火归原,导浮越之阳气归于命门,益火消阴㊂若患者出现咽痛㊁牙龈肿痛㊁痤疮等阳气不敛㊁虚火上冲之象,常用砂仁㊁肉桂以收敛阳气,纳气归肾,引火归原㊂白芍味酸能敛,敛降甲木胆火,使相火归位㊂‘本草求真“曰: 气之盛者,必赖酸为之收,故白芍号为敛肝之液,收肝之气,而令气不妄行也㊂[9] 朱章志常使用白芍以补肝之体㊁助肝之用,收敛肝气,肝平则郁气自除,火热自消㊂山萸肉秘精气㊁敛阳气,使龙雷之火归于水中㊂朱章志常用山萸肉收敛正气,遇汗出多者,常重用以固涩敛汗㊂泽泻能泻能降,能入肾泻浊,开气化之源,泻浊以利扶正,又能降气而引火下行㊂朱章志常用泽泻打通西方潜藏之要塞[10],在温阳之品中加入泽泻,利于阳气潜藏,使孤阳有归㊂2.1.3㊀填补阴精,以滋化源㊀‘素问㊃金匮真言论篇“提到: 夫精者,身之本也㊂ 精 是人体生命活动的物质基础,能化气生髓,濡养脏腑㊂人体之精禀受于父母,又由后天水谷之精不断充养,归藏于肾中㊂ 孤阴不生,独阳不长 ,无阳则阴无以生,无阴则阳无以化㊂肾乃水火之脏,阴精充足才能涵养坎中真火,使真阳固密于内,化生正气㊂朱章志常在秋冬之季嘱糖尿病患者进补阿胶等血肉有情之品填补肾精㊂肾主封藏,秋冬进补使肾精充养,以滋阳气化生之源㊂阿胶用黄酒烊化,既能祛除阿胶之腥,又能借黄酒通行之性解阿胶滋腻碍胃之弊,每日少量服用,以有形之精难以速生,填补肾精以缓补为要㊂除此之外,遣方用药时亦会注意顾护阴精,在使用温阳药的同时常常配伍山萸肉㊁白芍等养阴药,以防温燥伤阴之弊㊂2.2㊀祛邪2.2.1㊀外散寒水以运太阳㊀ 太阳为开 ,太阳乃三阳之表,巨阳也,其性开泄以应天,为祛邪之重要通道㊂在运气里,太阳在天为寒,在地为水,合而为太阳寒水㊂张仲景太阳病篇研究的是水循环过程,治太阳就是治水[11]㊂寒㊁水之邪闭郁在表,气血运行不畅,可见肌肤麻木不仁㊂邪气滞留太阳,阻碍阳气运行,当因势利导㊁开太阳之表以发汗,外散寒㊁水之邪㊂糖尿病患者正气亏虚为本,祛邪不能伤正,朱章志临床常用桂枝麻黄各半汤小发其汗,使玄府开张,邪有出路㊂桂枝麻黄各半汤乃发汗轻剂,为桂枝汤与麻黄汤相合而得,其中麻黄㊁桂枝㊁生姜㊁北杏发散宣肺以开皮毛,芍药㊁大枣㊁炙甘草酸甘化阴以益营,诸药相合,刚柔相济,祛邪而不伤正㊂邪去正安,阳气运行通畅,水液代谢复常则阳气自充,而无寒水之扰㊂若寒邪较重可用三拗汤,此为麻黄汤去桂枝而成,功善开宣肺气,疏散风寒,因去辛温之桂枝发汗力不及麻黄汤,祛邪而不伤正㊂2.2.2㊀下利水湿以健少阴㊀少阴乃水火交会之脏,元气之根,人身立命之本㊂‘医理真传“提到: 坎中真阳,一名龙雷火,发而为病,一名元阳外越,一名孤阳上浮,一名虚火上冲㊂此际之龙,乃初生之龙,不能飞腾而兴云布雨,惟潜于渊中,以水为家,以水为性,遂安其在下之位㊂水盛一分龙亦盛一分,水高一尺龙亦高一尺,是龙之因水盛而游 [12]㊂阴盛051中国中医基础医学杂志Journal of Basic Chinese Medicine㊀㊀㊀㊀㊀㊀2021年1月第27卷第1期January2021Vol.27.No.1则阳衰,水湿之邪泛滥,则龙雷之火因而飞越在外㊂叶天士深谙张仲景之理,提到 通阳不在温,而在利小便 [10,13],通过利小便的方法,使水湿之邪从下而解,阳气运行通道无水湿之邪阻碍,则阳气无需温养而自通,水盛得除则真龙亦安其位㊂朱章志常用五苓散㊁真武汤下利水湿,以复阳气之通达,少阴之健运㊂五苓散具有通阳化气利水之效,治疗膀胱气化不利形成的蓄水证㊂方中猪苓㊁茯苓㊁泽泻导水湿之邪下行;白术健脾燥湿,杜绝生湿之源;桂枝助膀胱气化,通阳化气行水又通气于表,使全身在表之湿邪皆得解,五药合用,膀胱气化复常,水道通调使小便得利,水湿得出㊂真武汤为治疗少阴阳虚㊁水气泛滥之主方,方中附子振奋少阴阳气,使水有所主;白术㊁茯苓健脾制水;生姜助附子敷布阳气,宣散水气;芍药利小便,制附㊁姜之燥,五味相合共奏温阳利水之功㊂2.2.3㊀开郁逐寒以畅厥阴㊀肝为将军之官,肝气主动主升发,能统帅兵马,捍卫君主㊂厥阴肝经,体阴用阳,内寄相火,相火敷布阳气,祛阴除寒,是祛邪的先锋主力军㊂朱章志常用吴茱萸汤祛除厥阴肝经之寒邪,恢复肝经阳气之运行㊂方中吴茱萸辛苦而温,芳香而燥,‘本草汇言“曰: 开郁化滞,逐冷降气之药 [14],能温胃暖肝,降浊阴止呕逆,为治疗肝寒之要药㊂配以生姜温胃散寒,佐以人参㊁大枣健脾益气补虚,全方散寒与降逆并施,共奏暖肝温胃㊁降逆止呕之效㊂‘素问㊃至真要大论篇“说: 帝曰:厥阴何也?岐伯曰:两阴交尽也㊂ 物极必反,重阴必阳㊂厥阴为阴尽阳生之脏,足厥阴肝经与足少阳胆经互为表里,若出现肝气不疏㊁枢机不利㊁气郁化火,朱章志常用小柴胡汤和畅枢机,开郁以复气机调达㊂方中柴胡疏泄肝胆之气;黄芩清泄胆火,一疏一清,气郁通达,火郁得发;生姜㊁半夏和胃降逆;人参㊁大枣㊁炙甘草固护中宫,全方寒温并用㊁补泻兼施,以复厥阴疏泄之职,使气机和畅㊁阳气运行有序㊂3㊀典型病案患者杨某,女,65岁,2017年3月10日初诊:2型糖尿病病史6年余,症见疲乏,双下肢轻度浮肿,下肢冰凉,背部易汗出,口苦口干,偶有腰膝酸软,纳眠可,二便调,舌淡暗,苔黄腻,脉沉细㊂辅助检查示糖化血红蛋白10.8%,空腹血糖14.59mmol/L,总胆固醇6.38mmol/L,甘油三酯3.66mmol/L,低密度脂蛋白胆固醇4.34mmol/L㊂西医诊断2型糖尿病㊁高脂血症,治疗给予门冬胰岛素30(早餐前22u晚餐前20u)控制血糖,阿托伐他汀钙片(20mg, qn)调脂㊂中医诊断消渴病,少阴阳虚寒湿证㊂患者少阴阳气衰微不足以养神,固见疲乏;腰为肾之府,少阴阳虚则见腰膝酸软,阳虚寒盛则见下肢冰凉;背部正中乃督脉运行之所,阳气虚衰无以固摄则见背部汗出;少阴阳虚不能主水,寒水泛滥则见双下肢浮肿;水湿内停有郁而化热之象,则见口苦口干㊁舌苔黄腻㊁舌淡暗,脉沉细为少阴阳虚寒湿之征,治以温阳散寒㊁利水除湿为法㊂方以扶正祛邪方合当归拈痛汤加减:炮附片10g(先煎1h),红参10g (另炖),干姜15g,白术30g,炙甘草15g,桂枝12 g,麻黄8g,生姜30g,猪苓10g,泽泻30g,茯苓30 g,白芍30g,酒萸肉45g,当归15g,茵陈10g,5剂水煎服,2d1剂,水煎至250ml,饭后分2次服用,次日复煎㊂方中以附子理中汤为底方温补中焦,散寒除湿;加桂枝㊁麻黄使寒湿之邪从皮毛而解;加五苓散通阳化气,使湿邪从下而出;生姜散寒除湿;白芍㊁酒萸肉收敛阳气,以助正气祛邪;当归活血利水;茵陈清热利湿㊂2017年3月24日二诊:患者双下肢浮肿减轻,疲乏较前好转,无口干口苦,无腰膝酸软,仍觉下肢冰凉,背部仍有汗出,动则尤甚,大便每日二行,质偏烂,舌淡暗,苔白腻,脉细㊂患者大便质烂,乃邪有出路,导水湿之邪从大便而解㊂患者无口干口苦,舌苔由黄腻转为白腻,知湿郁化热之象已除,遂去茵陈㊂仍觉下肢冰凉乃内有久寒,加制吴茱萸12g以散沉寒痼冷;上方加酒萸肉至60g以加强收敛阳气㊁固摄敛汗之效,加黄芪60g以健脾益气敛汗;加砂仁6g(后下)㊁肉桂3g(焗服)以加强收敛阳气㊁扶助正气之效,7剂水煎服,服法同前㊂2017年4月7日三诊:患者背部汗出减少,下肢转温,余症皆除,大便每日二行质软,舌淡红,苔薄白,脉细较前有力,继续服二诊方药5剂㊂后给予附子理中丸(每次8粒,每日3次)服用1个月巩固疗效㊂2017年11月17日复诊:患者上述症状皆除,纳眠可,二便调㊂复查糖化血红蛋白6.8%,空腹血糖6.5mmol/L,总胆固醇5.14mmol/L,甘油三酯1.65 mmol/L,低密度脂蛋白胆固醇2.43mmol/L㊂4㊀结语以往医家多以滋阴清热为法治疗糖尿病,通过长期的临床实践,朱章志不囿陈法,根据糖尿病患者当前之病因病机特点,运用扶正祛邪法治疗糖尿病,通过顾护太阴㊁扶助阳气㊁收敛阳气,祛除寒水湿之邪气,恢复阳气运行之通畅,为糖尿病的治疗提供新思路㊂参考文献:[1]㊀WANG L GAO-P-ZHANG-M,et al.Prevalence and EthnicPattern of Diabetes and Prediabetes in China in2013[J].JAMA,2017,317(24):2515-2523.[2]㊀谭宏韬,刘树林,朱章志,等. 首辨阴阳,再辨六经 论治惠州地区2型糖尿病的临床观察[J].中华中医药杂志,2018,33(9):4240-4244.(下转第181页)offspring of sleep-deprived mice[J].Psychoneuroendocrinology,2009,35(5):775-784.[9]㊀覃甘梅,覃骊兰.心肾不交型失眠动物模型研究进展[J].中华中医药杂志,2018,33(1):229-231.[10]㊀吕志平,刘承才.肝郁致瘀机理探讨[J].中医杂志,2000,41(6):367-368.[11]㊀游秋云,王平,田代志,等.老年肝郁失眠证候大鼠模型的建立和评价[J].中国实验方剂学杂志,2013,19(2):222-225. [12]㊀唐仕欢,杨洪军,黄璐琦. 以方测证 方法应用的反思[J].中国中西医结合杂志,2007,27(3):259-262.[13]㊀卢岩,刘振华,于晓华,等.疏肝调神针法针刺对睡眠剥夺模型大鼠神经递质的影响[J].山东中医杂志,2017,36(4):322-325. [14]㊀YANG CR,SEAMANS JK,GORELOVA N.Developing aneuronal model for the pathophysiology of schizophrenia based onthe nature of electrophysiological actions of dopamine in theprefrontal cortex[J].Neuropsychopharmacology,1999,21(2):161-194.[15]㊀何林熹,诸毅晖,杨翠花,等.失眠肝郁化火证大鼠模型的建立及其评价[J].中华中医药杂志,2018,33(9):3890-3894. [16]㊀李越峰,徐富菊,张泽国,等.四逆散对大鼠睡眠时相影响的实验研究[J].中国临床药理学杂志,2014,30(10):936-938. [17]㊀张晓婷,刘文超,刘俊昌,等.电击法建立SD大鼠焦虑型心理应激-失眠模型的研究[J].现代中西医结合杂志,2018,27(30):3316-3319.[18]㊀钱伯初,史红,郑晓亮.新的失眠动物模型研究概述[J].中国新药杂志,2008,17(1):1-4.[19]㊀朱洁,申国明,汪远金,等.肝郁证失眠大鼠模型的建立与评价[J].中医杂志,2011,52(8):689-692.[20]㊀刘倩,李蜀平,廖磊,等.调和肝脾方治疗失眠的实验研究[J].北京中医药,2018,37(8):768-770.[21]㊀全世建,焦蒙蒙,黑赏艳,等.交泰丸对睡眠剥夺大鼠下丘脑Orexin A及γ-氨基丁酸的影响[J].广州中医药大学学报,2015,32(1):103-105.[22]㊀KOBAN M,SWINSON KL.Chronic REM-sleep deprivation ofrats elevates metabolic rate and increases UCP1gene expressionin brown adipose tissue[J].Am J Physiol Endocrinol Metab,2005,289(1):68-74.[23]㊀赵俊云,杨晓敏,胡秀华,等.失眠动物模型HPA轴和表观遗传修饰的变化及交泰丸的干预作用[J].中医药学报,2018,46(4):19-21.[24]㊀GORGULU Y,CALIYURT O.Rapid antidepressant effects ofsleep deprivation therapy correlates with serum BDNF changes inmajor depression[J].Brain Res Bull,2009,80(3):158-162.[25]㊀BENCA RM,PETERSON MJ.Insomnia and depression[J].Sleep Med,2008,9(1):S3-S9.[26]㊀郜红利,涂星,卢映,等.心肾不交所致失眠大鼠模型[J].中成药,2014,36(6):1138-1141.[27]㊀杨钰涵,孙雨,王珺,等.中医病证相符的大鼠心肾不交失眠模型的建立及其血清代谢组学研究[J].中国中药杂志,2020,45(2):383-390.[28]㊀石皓月,鲁艺,李钰昕,等.中药治疗对氯苯丙氨酸失眠模型大鼠影响的基础研究进展[J].中国医药导报,2018,15(11):33-36.[29]㊀全世建,何树茂,钱莉莉.交泰丸交通心肾治疗失眠作用机理研究[J].辽宁中医药大学学报,2011,13(8):12-14. [30]㊀GULEC M,OZKOL H,SELVI Y,et al.Oxidative stress inpatients with primary insomnia[J].Pro NeuropsychopharmacolBiol Psychiatry,2012,37(2):247-251.[31]㊀ZHANG H,CAO D,CUI W,et al.Molecular bases ofthioredoxin and thioredoxin reductase-mediated prooxidant actionsof(-)-epigallocatechin-3-gallate[J].Free Radic Biol Med,2010,49(12):2010-2018.[32]㊀谢光璟,刘源才,胡辉,等.基于Trx系统介导的抗氧化应激探讨天王补心方对失眠模型大鼠的干预作用[J].时珍国医国药,2019,30(4):805-808.[33]㊀黄攀攀,王平,李贵海,等.老年阴虚失眠动物模型的建立与评价[J].中华中医药学刊,2010,28(8):1719-1723.[34]㊀XIONG L,HUANG XJ,SONG PX.The experiment ofstudymodel of Deficiency of yin Insomnia by Yangyin anshenkoufuye[J].Chin J Pract Chin Mod Med,2005,18(18):1187-1188.[35]㊀韦祎,唐汉庆,李克明,等.脾阳虚证失眠大鼠模型的建立和附子理中汤的干预效应[J].中国实验方剂学杂志,2013,19(16):289-292.[36]㊀王志鹏.桂枝甘草龙骨牡蛎汤对阳虚证失眠大鼠脑内5-HT㊁NE含量的影响[D].南京:东南大学,2015.[37]㊀MURRAY NM,BUCHANAN GF,RICHERSON GB.InsomniaCaused by Serotonin Depletion is Due to Hypothermia[J].Sleep,2015,38(12):1985-1993.[38]㊀宋亚刚,李艳,崔琳琳,等.中医药病证结合动物模型的现代应用研究及思考[J].中草药,2019,50(16):3971-3978. [39]㊀李晓娟,白晓晖,陈家旭,等.中医动物模型研制方法及展望[J].中华中医药杂志,2014,29(7):2263-2266.[40]㊀刘臻,谢晨,赵娜,等.失眠动物模型的制作与评价[J].中医学报,2013,28(12):1846-1848.收稿日期:2020-05-18(上接第151页)[3]㊀司芹芹,牛晓红,杨海卿,等.温阳益气养阴活血方治疗2型糖尿病肾病的临床疗效[J].中华中医药学刊,2018,36(3):703-705.[4]㊀郭仪,石岩.中药复方治疗糖尿病大血管病变临床疗效及对血糖㊁血脂影响的系统评价[J].中华中医药学刊,2017,35(6):1369-1375.[5]㊀方春平,刘步平,朱章志.‘伤寒论“中 胃气 思想在病脉辨证中的运用[J].浙江中医药大学学报,2014,38(8):948-950.[6]㊀黄元御.四圣心源[M].北京:中国中医药出版社,2009:24.[7]㊀朱章志,林明欣,樊毓运.立足 阳主阴从 浅析糖尿病的中医治疗[J].江苏中医药,2011,43(4):7-8.[8]㊀缪希雍.本草经疏[M].北京:中国医药科技出版社,2011:56.[9]㊀黄宫绣.本草求真[M].北京:中国中医药出版社,2008:132.[10]㊀林明欣,裴倩,朱章志.浅析 通阳不在温,而在利小便 [J].中医杂志,2011,52(19):1705-1706.[11]㊀刘力红.思考中医[M].桂林:广西师范大学出版社,2006:457.[12]㊀郑寿全.医理真传[M].北京:中国中医药出版社,2008:3.[13]㊀刘涛,张毅,李娟,等.结合‘伤寒论“探讨 通阳不在温而在利小便 [J].中国中医药信息杂志,2017,24(9):106-107.[14]㊀倪朱谟.本草汇言[M].北京:中医古籍出版社,2010:87.收稿日期:2020-04-27。
地质专业英语词汇C

地质专业英语词汇C c battery 栅偏压电池cable belt 缆摔带运输机cable belt conveyor 缆摔带运输机cable drilling 钢丝绳钻进cable reel 缆轴cable splicing 电缆编接cable tool 绳岁钻具cable tool drilling 顿钻钻井cabrerite 镁镍华cacheutaite 硒铜铅矿cadastral map 地籍图cadmium 镉cage winding 罐笼提升cahnite 水砷硼钙石cainosite 钙钇铒矿cainozoic era 新生代caisson 沉箱cake 块caking ability 粘结度caking coal 粘结煤caking index 粘结指数caking property 粘结性calafatite 水钾铝矾calamine 异极矿calaverite 磅金矿calc alkali granite 钙碱花岗岩calc alkali magma 钙碱岩浆calc alkali rock series 钙碱岩系calc alkali series 钙碱系calc alkaline rock 钙质碱性岩calc aphanite 钙质隐晶岩calc spessartine 钙锰铝榴石calcareous dolomite 钙质自云岩calcareous marl 灰质泥灰岩calcareous ooze 钙质软泥calcareous schist 钙质片岩calcareous sediments 钙质沉积物calcareous soil 石灰性土壤calcareous sponges 石灰海绵类calcareous tufa 石灰华calccrust 钙质层calcian dolomite 钙质白云岩calciferous 钙质的calcification 钙化calcination 煅烧calcio ancylite 钙菱锶铬矿calciobiotite 钙黑云母calcioferrite 钙磷铁矿calciphilous plant 喜钙植物calciphobe plant 厌钙植物calciphyre 斑花大理石calcite 方解石calcite twinning 方解石双晶calcitic dolomite 方解白云岩calcium 钙calcium base mud 钙基泥浆calcomalachite 钙孔雀石calcrete 灰质结砾岩calculating rule 计算尺caldera 破火山口caldera lake 巨火山口湖caldron 海釜caledonian folding 加里东褶皱caledonian orogeny 加里东造山运动caledonite 铅绿矾calibration 校准calibration curve 校准曲线calibration of seismograph 地震仪校准caliche 钙质层californium 锎caliper 井径仪caliper logging 井径测量callovian 卡洛维阶calomel 甘汞calomel electrode 甘汞电极calorific value of coal 发热量calyx 萼calyx lobe 萼片cambrian system 寒武系campanian 坎帕阶camptonite 斜闪煌岩camsellite 硼镁石canada balsam 加拿大屎canadite 钢霞正长岩canal 运河canal structure 渠道构造cancrinite 钙霞石cancrinite syenite 钙霞正长岩canfieldite 黑硫银锡矿canine 大齿cannel coal 烛煤cannizzarite 辉铅铋矿cantalite 疗松脂岩cantonite 方铜蓝canyon 峡谷cap 罩盖cap rock 盖保岩cape 岬capillaceous 毛状的capillar migration 毛细管迁移capillarity 毛细管现象capillary 毛状的capillary fringe 毛细管酌带capillary water 毛细管水capnite 铁菱锌矿caporcianite 红粒浊沸石cappelenite 硼硅钡钇矿capping 复盖岩层capstan 绞盘capture cross section 俘获截面captured river 袭夺河car pusher 推车机caracolite 氯铅芒硝carat 克拉carbargillite 碳质页岩carboapatite 碳酸磷灰石carbohydrate 碳水化合物carbon 碳carbon compound 碳化合物carbon content in coal 煤中碳carbon cycle 碳化循环carbon dioxide 二氧化碳carbonaceous 碳质的carbonaceous chondrite 碳质球粒陨石carbonaceous shale 碳质页岩carbonado 黑金刚石carbonate 碳酸盐carbonate apatite 碳酸磷灰石carbonate deposits 碳酸盐质沉积carbonate rock 碳酸盐岩carbonate sediments 碳酸盐质沉积carbonated spring 碳酸泉carbonated water 碳酸水carbonation 碳酸盐化carbonatite 碳酸盐岩carbonatite magma 火成碳酸盐岩浆carbonatization 碳酸盐化carbonic acid 碳酸carbonic period 石炭纪carboniferous 石炭纪的carboniferous period 石炭纪carbonification 碳化酌carbonization 碳化酌carbonous 含碳的carborundum 金刚砂carburane 碳铀矿cardiophyllous 心形叶的carina 龙骨carinate 龙骨状的carious 虫牙状的carlsbad twin 卡斯巴双晶carmeloite 伊丁玄武岩carminite 砷铅铁矿carnallite 光卤石carnegieite 三斜霞石carnian 卡尼克阶carnotite 钾钒铀矿carphosiderite 草黄铁矾carrier 载体carrollite 硫铜钴矿carrying capacity 运载能力cartilage 软骨cartilage cell 软骨细胞cartilaginous fishes 软骨鱼类cartilaginous tissue 软骨细胞cartogram 统结计图cartography 绘图法caryinite 砷锰铅矿caryocerite 褐稀土矿caryopilite 肾硅锰矿cascade fold 叠褶cascadite 橄辉云煌岩casing 套管casing joint 套管联接casing line 下套管钢丝绳casing pipe 套管casing shoe 套管鞋cassiterite 锡石cast 铸型castanite 褐铁矾castellite 铝钛片晶石castings fecal pellets 粪化石cat's eye 猫眼石catabolism 分化酌cataclasis 碎裂酌cataclasite 碎裂岩cataclastic 碎裂的cataclastic cleavage 碎裂劈理cataclastic metamorphism 碎裂变质cataclastic rock 碎裂岩catagenesis 退化catalysis 催化cataphoresis 电泳cataphorite 红钠闪石catapleite 钠锆石catastrophic theory 灾变论catastrophism 灾变论catawberite 滑石磁铁岩catchment 集水区catchment area 汇水面积category 类category of reserves 储量级别cathead 吊锚架cathodic protection 阴极保护cation exchange 阳离子交换catlinit 烟斗泥caudal fin 尾caulorrhizous 茎状根的caustic alkali 苛性碱caustic metamorphism 腐蚀变质酌caustobiolite 可燃性生物岩cave 洞cave in 塌落cave sediments 洞穴沉积cavern 岩洞cavern water 溶洞水cavernous 洞穴状的cavernous body 海绵体cavernous rock 孔状岩石cavernous structure 洞穴状构造caving 塌落caving line 崩落线cavity 腔cavity filling deposit 洞穴填充矿床cay 砂礁cebollite 纤维石cedar forest 雪松林cedarite 松脂石cegamite 水锌矿ceilometer 测云仪celadonite 绿鳞石celestine 天青石celestite 天青石cell 细胞;电池cell division 细胞分裂cellular 细胞状的cellular dolomite 细胞状白云岩cellular tissue 细胞组织cellulose 纤维素celom 体腔celsian 钡长石cement 胶结物cement bond 水泥胶结cement bond logging 固井测井cement grout 水泥浆cement mortar 水泥砂浆cement seal 水泥封闭cement slurry 水泥浆cement substance 粘合质cement water factor 水灰比cement water ratio 水灰比cementation 胶结cementation borehole 水泥灌浆钻孔cementation zone 胶结带cementing 水泥灌浆cementum 水泥质cenobiosis 群落生活cenogenesis 新生成因cenoman 赛诺曼cenomanian stage 赛诺曼cenophytic era 新生植物代cenosite 钙钇铒矿cenote 锅穴cenozoic 新生代cenozoic era 新生代center line 中线center of crystal lisation 晶核center of dispersal 分布中心center of pressure 压力中心center of symmetry 对称中心central body 中央体central cone 中央锥central cylinder 中心柱central dome 中央圆丘central eruption 中心喷发central plain 湖心平原central type volcano 中心式火山centrallassite 白硅钙石centrifugal pump 离心泵centrifugation 离心分离centrifuge method 离心分离法centripetal 向心状的centripetal elements 向心元素centroclinal dip 向心倾斜centroclinal fold 向心褶皱centrocline 向心倾斜centrosphere 地心圈cephalopoda 头足类cerargyrite 角银矿cerasite 樱石cerite 硅铈石cerium 铈cerolite 蜡蛇纹石cerussite 白铅矿cervantite 锑舣cervical region 颈部cesarolite 铅锰矿cesium 钯ceylonite 镁铁尖晶石chabasite 菱沸石chain 链;山脉chain compound 链化合物chain like veins 锁键状矿脉chain molecule 链型分子chain silicates 链硅酸盐chalcanthite 胆矾chalcedonite 玉髓chalcedony 玉髓chalcoalumite 铜茂chalcocite 辉铜矿chalcography 矿相学chalcolite 铜铀云母chalcomenite 蓝铜硒矿chalcophanite 黑锌锰矿chalcophile elements 亲铜元素chalcophyllite 云母铜矿chalcopyrite 黄铜矿chalcosiderite 磷铜铁矿chalcosine 辉铜矿chalcostibite 硫铜锑矿chalcotrichite 毛赤铜矿chalk 上白垩纪;白垩chalk ooze 白垩软泥chalmersite 方黄铜矿chalybite 菱铁矿chamber 室chamber of ore 矿袋chambered vein 囊状矿脉chamosite 鲕绿泥石chamotte 耐火粘土chamotte brick 粘土砖champion vein 巨矿脉change in type of geosyncline 地槽类型转化channel 槽水道channel cast 槽痕迹channel characteristics 河道特性channel precipitation 河道沉淀channel spring 沿河泉channel strait 海峡channel structure 沟渠构造character 形质character species 特罩characteristic curve 特性曲线characteristic hydrograph 特性水位图characteristic radiation 特性辐射characteristic species 特罩characteristic x rays特性x 射线charcoal 木炭charge 电荷charge transfer 电荷转移chart 图chassignite 纯橄无球粒陨石chatham emerald 人造翡翠chathamite 砷镍铁矿chatoyancy 光条chatoyant 光条chatter mark 颤动擦痕chatter marks 慧check borehole 检验钻孔check sample 校对试样check valve 防逆阀chela 螯chelate 螯形化合物chelating agent 螯合剂chelation 螯合酌chelatometric titration 螯合滴定chelatometry 螯合滴定chemical affinity 化学亲合势chemical atomic weight scale 化学原子量标度chemical bond 化学键chemical bond energy 化学键能量chemical composition 化学成分chemical conditioning of mud 泥浆的化学处理chemical constitution 化学构造chemical deposit 化学沉积chemical equilibrium 化学平衡chemical equivalent 化学当量chemical erosion 化学侵蚀chemical evolution 化学进化chemical fossil 化学化石chemical mineralogy 化学矿物学chemical mobility 化学怜性chemical oxygen demand 化学需氧量chemical potential 化学势chemical precipitation 化学沉积chemical precipitation ore deposit 化学沉淀矿床chemical property 化学性质chemical prospecting 化探chemical reaction 化学反应chemical reduction 化学还原chemical remanent magnetization 化学残余磁化chemical sedimentary deposit 化学沉积矿床chemical sediments 化学沉积物chemical stratification 化学成层chemical types of geothermal fluids 地热铃的化学的类型chemical weathering 化学风化chemisorption 化学吸附chemometamorphism 化学变质chenevixite 绿砷铁铜矿chernozem 黑土cherokine 乳白铅矿chert 燧石chessylite 蓝铜矿chestnut soil 栗土chevkinite 硅钛铈钇矿chevron fold 尖顶褶皱chevron mark 尖楞刻痕chiastolite 空晶石chileite 智利石chillagite 钨钼酸铅矿chiltonite 葡萄石chimney 管状矿体china clay 瓷土chinkolobwite 硅镁铀矿chip 碎片chisel bit 冲唤钻头chisel edge 凿切削chitin 甲壳质chiviatite 硫铅铋矿chladnite 顽无球陨石chloanthite 砷镍矿chlopinite 赫洛宾矿chlorapatite 氯磷灰石chloric acid 氯酸chloride 氯化物chlorination 氯化酌chlorine 氯chlorinity 氯度chlorite 绿泥石chlorite schist 绿泥片岩chloritization 绿泥石化chloritoid 硬绿泥石chloritoid schist 硬绿泥片岩chlormanganokalite 钾锰盐chloroform bitumen 氯仿沥青chloromagnesite 氯镁石chloromelanite 暗绿玉chloromelanitite 浓绿玉岩chlorometry 氯量滴定法chloropal 绿蛋白石chlorophaeite 蠕绿泥石chlorophane 绿萤石chloroplast 叶绿体chlorosity 体积氯度chlorospinel 绿尖晶石chlorothionite 钾氯胆矾chomata 隔壁褶皱chondrin 软骨胶chondrite 球粒陨石chondrocranium 软骨颅chondrodite 粒硅镁石chondrule 陨石球粒chondrus 陨石球粒chonolite 畸形岩盘chordates 脊睡物门chorismite 混合岩chorologic unit 分布单位christianite 钾沸石chromatic aberration 色像差chromatographic contact print method 色谱接触印相法chromatography 色层分离法chrome 铬chrome diopside 铬透辉石chrome sand 铬砂chromic acid 铬酸chromite 铬铁矿chromite deposit 铬铁矿床chromium 铬chromotropic acid 铬变酸chrompicotite 硬铬尖晶石chronolithologic unit 年代地层单位chronologic scale 地质年代表chronology 年代学chronostratigraphic unit 年代地层单位chronostratigraphy 年代地层学chronozone 时带chrysoberyl 金绿宝石chrysocolla 硅孔雀石chrysolite 贵橄榄石chrysoprase 绿玉髓chrysotile 纤维蛇纹石chrysotile asbestos 纤维蛇纹石churchite 水磷铈矿churn drill 钢丝绳钻机churn drilling method 冲魂井方法chute 斜槽ciliary 纤毛的ciliates 纤毛虫类ciminite 正边粗面粗玄岩cimmerian 误里阶cimolite 水土cinder 火山渣cinder cone 火山渣锥cinerite 火山凝灰岩cinnabar 辰砂ciplyite 磷硅钙石cipolino 云母大理岩cipollin 云母大理岩cipw classification cipw 分类circle of influence 影响圈circular structure 环状构造circulating mud 循环泥桨circulating water 循环水circulation 循环circulation drilling 环陵井circulation index 环粮数circum pacific 环太平洋的circum pacific calc alkaline petrographic province 环太平洋钙碱性岩石区circum pacific geothermal belt 环太平洋地热带circum pacific island arc 环太平洋岛弧circum pacific seismic zone 环太平洋地震带circum pacific volcanic belt 环太平洋火山带circumdenudation 环蚀circumerosion 环蚀circumference 圆周circumoceanic andesite 环洋安山岩cirque 环谷cirrolite 黄磷钙铝石cistern 蓄水池citrate 柠檬酸盐citric acid 柠檬酸citrine 黄晶civil engineering 土木工程clarain 亮煤clarite 亮煤clarke number 克拉克值clarkeite 水钠铀矿clarodurite 微亮暗煤clasolite 碎屑岩class 纲classification 分类classification of organisms 生物分类classifier 分级机classify 分类clast 碎屑物clastic 碎屑状的clastic breccia 碎屑角砾岩clastic deformation 碎屑变形clastic deposits 碎屑沉积物clastic dike 碎屑岩墙clastic ratio 碎屑比clastic rock 碎屑岩clastic sediments 碎屑沉积clastic structure 碎屑构造clastic wedge 碎屑楔状体clastics 碎屑物clastocrystalline 碎屑结晶质clastogranitic 碎屑花岗质的clathrate texture 格子状组构claudetite 白砷石clausthalite 硒铅矿clavalite 哑铃晶clavate 棒状的claviform 棒状的clavoid 棒状的clay 粘土clay coating 泥糊clay deposit 粘土矿床clay desert 粘土荒漠clay film 泥糊clay gall 粘土片clay gouge 断层泥clay ironstone 泥铁岩clay mineral 粘土矿物clay plug 粘土塞子clay skin 泥糊clay soil 粘土质土壤clay stone 粘土岩clay vein 粘土脉clayey 泥质的clayey sand 粘土质砂claying of soil 土壤粘化clayish 泥质的claypan 隔水粘土层clayzation 粘土化clean sand 净砂层cleaning 精选cleaning bottom of hole 扫孔clear relief 显地形clearance 间隙clearing basin 沉积池cleat 割理cleavage 解理cleavage face 劈理面cleavage fan 扇状劈理cleavage fold 劈理褶皱cleavage form 劈理形cleavage plane 劈理面cleave 劈开解理cleavelandite 叶钠长石cleiophane 纯闪锌矿cliff 悬崖cliff glacier 悬崖冰川cliftonite 方晶石墨climate 气候climatography 气候学climatostratigraphy 气候地层学climax 演替顶极climbingplant 绕攀植物clingmanite 珍珠云母clinker 熔块clinkstone 响岩clino axis 斜轴clino diagonal axis 斜轴clino prism 钭轴柱clino pyramid 斜轴锥clino unconformity 斜交不整合clinochlore 斜绿泥石clinoclase 光线矿clinoclasite 光线矿clinocrocite 铁钾茂clinodome 斜轴坡面clinoenstatite 斜顽辉石clinoferrosilite 斜铁辉石clinographic projection 斜射投影clinohedrite 斜晶石clinohumite 钭硅镁石clinohypersthene 钭柴苏辉石clinometer 地质罗盘clinopinacoid 斜轴面clinoptilolite 斜发沸石clinopyroxene 斜辉石clinounconformity 斜交不整合clinozoisite 斜帘石clintonite 绿脆云母clitellum 环带clockwise rotation 顺clockwise shearing 顺时针扭动clod 土粒cloddy 团块状的close packed structure 密集结构closed basin 封合盆地closed community 闭锁植物群落closed fault 闭合断层closed fold 闭合褶皱closed joint 闭式节理closed system 封闭系统closed tube analysis 闭管分析closest packing 最密充填closing error 闭合误差closterite 库克油页岩cloudburst 大暴雨club mosses 石松类clue method 重点区法cluster 群;串coacervate 团聚体coacervation 凝聚coadaptation 互相适应coagulant 凝结剂coagulation 凝聚coagulative power 凝结力coal 煤coal accumulating basin 聚煤盆地coal accumulating palaeostructure 聚煤古构造coal accumulating process 聚煤酌coal analysis 煤分析coal ash fusion temperature 煤灰熔点coal ball 煤层石球coal basin 煤盆地coal bearing formation 含煤地层coal bearing property 含煤性coal bearing ratio 含煤系数coal carbonization 煤之碳化coal deposits 煤矿床coal diagenesis 煤成岩酌coal district 煤产地coal dressing 选煤coal field 煤田coal field gas 煤田气coal for gasification 气化用煤coal forming period 聚煤期coal forming process 成煤酌coal gas 煤气coal geology 煤炭地质学coal measures 煤系coal metamorphism 煤变质酌coal mine 煤矿coal miner 煤矿工coal mining 采煤coal oil mixture 煤焦油混合物coal petrology 煤岩学coal preserving structure 贮煤构造coal property 煤质coal reserves 煤储量coal seam 煤层coalescing alluvial fan 复合冲积扇coalification 煤化酌coalification jump 煤化酌跃变coalman 煤矿工coaly 含煤的coarse grained texture 粗粒结构coarse sand 粗砂coarse soil 大粒土壤coarsness 粗粒度coast of dalmatian type 达尔马堤亚式海岸coast of elevation 隆起海岸coastal aquifer 滨海含水层coastal cave 海岸洞穴coastal land form 海岸地形coastal plain 海滨平原coastal sand dune 海岸沙丘coastal terrace 海岸阶地coastal vegetation 海滨植被coastline 海岸线coating 覆盖层cobalt 钴cobalt pyrite 硫钴矿cobaltine 辉钴矿cobaltite 辉钴矿cobaltocalcite 钴方解石cobaltomenite 硒钴矿cobaltsmithsonite 钴菱锌矿cobble stone 中砾石coblenzian stage 科布林茨阶coccinite 硒汞矿coccolite 粒辉石coccolith ooze 颗石藻软泥coccolithes 颗石藻coccyx 尾骨cockade ore 环状矿石cockade structure 鸡冠构造cockpit 灰岩盆地codazzite 菱钙铈矿code minier 矿山法则code of stratigraphic nomenclature 地层命名法规coeffcient of volume compressibility 体积压缩系数coefficient 系数coefficient of compressibility 压缩系数coefficient of consolidation 固结系数coefficient of discharge 量系数coefficient of elasticity 弹性系数coefficient of expansion 膨胀系数coefficient of friction 摩擦系数coefficient of permeability 渗透系数coefficient of relaxation 松弛系数coefficient of restitution 抗冲系数coefficient of safety 安全系数coefficient of uniformity 均匀系数coefficient of variation 变化系数coefficient of variation of grade 品位变化系数。
镉暴露诱发2型糖尿病机制研究进展
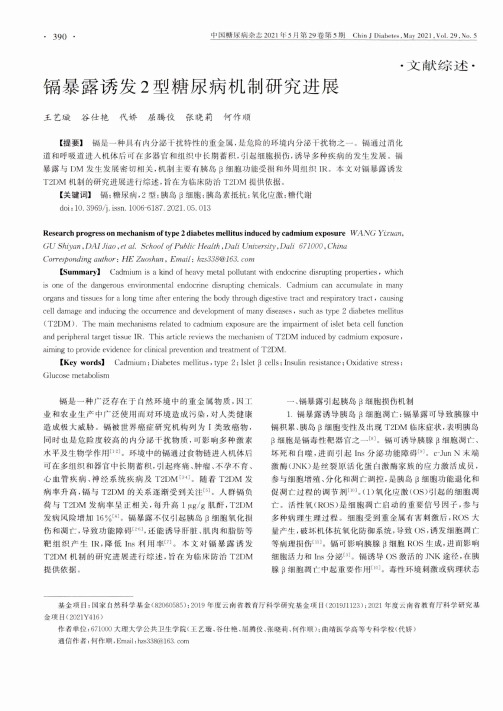
•390•中国糖诚病杂志2021 年5 月第29 卷第5 期Chin J Diabetes,Ma y 2021 ,Vol. 29, No. 5•文献综述•镉暴露诱发2型糖尿病机制研究进展王艺璇谷仕艳代娇屈腾佼张晓莉何作顺【提要】镉是一种具有内分泌干扰特性的重金属,是危险的环境内分泌干扰物之一。
镉通过消化道和呼吸道进入机体后可在多器官和组织中长期蓄积,引起细胞损伤,诱导多种疾病的发生发展。
镉暴露与D M发生发展密切相关,机制主要有胰岛卩细胞功能受损和外周组织IR。
本文对镉暴露诱发T2D M机制的研究进展进行综述,旨在为临床防治T2D M提供依据。
【关键词】镉;糖尿病,2型:胰岛卩细胞:胰岛素抵抗;氧化应激;糖代谢doi: 10. 3969/j. issn. 1006-6187. 2021.05.013Research progress on mechanism of type 2 diabetes mellitus induced by cadmium exposure WANG Yixuan,GU Shiyan, DAI Jiao ,et al. School o f P ublic Health,Dali University, Dali 671000,ChinaCorresponding author:HE Zuoshun, Email ••**************【Summary】Cadmium is a kind of heavy metal pollutant with endocrine disrupting properties,whichis one of the dangerous environmental endocrine disrupting chemicals. Cadmium can accumulate in manyorgans and tissues for a long time after entering the body through digestive tract and respiratory tra c t, causingcell damage and inducing the occurrence and development of many diseases, such as type 2diabetes mellitus(T2D M). The main mechanisms related to cadmium exposure are the impairment of islet beta cell functionand peripheral target tissue IR. T his article reviews the mechanism of T2DM induced by cadmium exposure,aiming to provide evidence for clinical prevention and treatm ent of T2DM.【Key words】Cadmium ; Diabetes m ellitus, type 2 ;Islet p cells ; Insulin resistance ; Oxidative stress ;Glucose metabolism镉是一种广泛存在于自然环境中的重金属物质,因工 业和农业生产中广泛使用而对环境造成污染,对人类健康造成极大威胁。
顶复门原虫磷脂酶A_(2)蛋白家族研究进展

functions of phospholipase A2,were reviewed in order to provide a theoretical basis for the prevention and treatment of protozoal disease and drug development.
摘 要 :顶 复 门 原 虫 属 于 专 性 细 胞 内 寄 生 的 单 细 胞 真 核 生 物 ,其 中 多 种 属 于 重 要 病 原 ,影 响 人类 的健 康 和 畜 牧 业 的 发 展 。 已 有 研 究 表 明 ,磷 脂 酶 A 2 为 顶 复 门 原 虫 发 病 机 制 中 的 关 键 酶 ,主 要 与 顶 复 门 原 虫 入 侵 、膜 重 塑 等 有 关 ,可 以 影 响 寄 生 虫 毒 力 和 疾 病 进 展 ,具 有 重 要 的 生 物 学 意 义 。 而 脂 肪 滋 养 蛋 白 磷 脂 酶 A 2(P L P )家 族 基 因 结 构 域 与 细 菌 P L P 相 似 性 较 高 ,与 真 核 生 物 P L P 存 在 显 著 差 异 ,成 为 研 究 抗 顶 复 门 原 虫 药 物 靶 点 的 热点 。本 文 就 近 年 来 磷 脂 酶 A 2 家 族 中 的 关 键 酶 P L P 及磷脂酶八2 的生物学功能在顶复门原虫上研究进展进 行 综 述 ,以 期 为 寄 生 原 虫 病 的 防 治 提 供 理 论 依 据 。
农业生态系统节肢动物功能生物多样性的调查与分析

中文摘要
本项研究选择稻田生态系统、蔬菜生态系统和 果园生态系统作为农业生态系统的子系统,调 查这些系统在有机与传统农业耕作方式条件下 节肢动物功能生物多样性,了解植食功能、寄 生功能、捕食功能和中性功能生物多样性的代 表类群及其指示物种,研究其食物链主要组成 物种的生命表与取食率,分析群落成分和物种 丰富度以及耕作方式对功能生物多样性的影响。 其结果将为解释上述生态系统的生态过程、开 发农作物病虫害持续控制技术提供理论和实验 依据。
生物多样性与生态系统功能之 间关系的研究
生态学上的理论研究和实验研究已趋向于生物多样性 与生态系统功能之间关系的研究。 陆地草原的研究结果表明,草原生态系统中的植物物 种的数量和性质对生态系统的功能有很强的影响 (Hooper 等 2005)。 微生物学的实验结果显示,在多水平的食物网中,不 断变化的生物多样性对生态系统的稳定性有明显的影 响(Petchey 等 2002)。 多营养水平研究的结果指出,相对于食饵物种的弱组 合来说,多种多样的食饵物种组合对于从上而下的控 制更具抵抗性,更能完善利用它们自己的资源,增加 消费者的适合度;而捕食物种的多样性既能增加也能 降低从上而下的控制(Lin et al.2009)。
功能生物多样性的研究
Jani Heino(2005)研究了大型节肢动物沿溪流生态梯 度变化的功能生物多样性,回归分析和多余分析 (redundency analysis)结果显示,功能生物多样性 的变化不仅与局部环境因子有关系,而且与环境变量 的空间型(spatial patterning of environmental variables)和空间梯度有关系。 M.Scherer-Lorenzen(2008)研究了功能多样性对实 验草地的分解过程的影响,结果指出,废弃物的分解 随物种功能组的增加而增加,而物种丰富度却对分解 没有影响,固氮的豆科植物能增加分解的速率。 John 等人(2009)使用功能多样性预测物种组合 (species combination)对第一生产力的超产效应。
猴头菌提取物对小鼠D-半乳糖衰老模型脑组织的抗氧化作用

猴头菌提取物对小鼠D-半乳糖衰老模型脑组织的抗氧化作用刘浩;李鸣皋;韩磊【期刊名称】《临床误诊误治》【年(卷),期】2011(24)7【摘要】Objective To explore the effects of Hericium extract on Antioxidative system in apolexis brain tissues in mice induced by D-galactose.Methods 60 seven or eight-week-old mice were chosen and divided into negative control group, positive control group, and high, medium and low dose of Hericium extract treatment group (12 in each).Positive control group, Vitamin EC mixture group and the 3 treatment groups were rendered to models by subcutaneous injection of D-galactose with a dosage of 100 mg/( kg · d).Meanwhile, the negative control group received subcutaneous injection of 0.9% sodium chloride.Then the high, medium and low dose of Hericium extract treatment group received Hericium extract with dosages of 1.5, 3.0, 4.5g/( kg · d) respectively.Both negative and positive control groups received distilled water with the same dosages as other 3 groups.The experiment continued for 4 weeks.Content of SOD and GSH-Px in brain tissues and MDA in brain tissues and blood serum were determined.Results The expression rates of SOD and GSH-Px in the 3 treatnent groups increased significantly after treatment, MDA content in the 3 treatment groups decreased significantly, and there were significant differences between thetreatment groups and positive group(P <0.01 ,P <0.05).Conclusion Hericium extract has significant antioxidative effect in apolexis brain tissues in mice induced by D-galactose.%目的探讨猴头菌提取物对D-半乳糖所致小鼠衰老模型脑组织的抗氧化作用.方法选择7~8周龄雄性小鼠60只,随机分为阴性对照组、阳性对照组及猴头菌提取物高、中、低剂量治疗组,每组12只.阳性对照组及3个治疗组按100 mg/(kg·d)皮下注射D-半乳糖造模,阴性对照组以相同方式予同剂量0.9%氯化钠注射液.造模后,猴头菌提取物低、中、高治疗组分别按1.5、3.0、4.5 g/(kg·d)予猴头菌提取物,阴性对照组和阳性对照组予同剂量蒸馏水,连续实验4周.检测小鼠脑组织中超氧化物歧化酶(SOD)、谷胱甘肽过氧化物酶(GSH-Px)和脑、血清中丙二醛(MDA)含量.结果经3个剂量猴头菌提取物治疗后,衰老小鼠脑组织中SOD、GSH-Px的表达显著升高,MDA含量显著下降,与阳性对照组比较差异有统计学意义(P<0.01,P<0.05).结论猴头菌提取物对D-半乳糖所致衰老小鼠脑组织具有明显抗氧化作用.【总页数】2页(P11-12)【作者】刘浩;李鸣皋;韩磊【作者单位】100048,北京,解放军海军总医院空潜科;100048,北京,解放军海军总医院空潜科;100048,北京,解放军海军总医院空潜科【正文语种】中文【中图分类】R-33【相关文献】1.平贝母多糖对D-半乳糖诱导衰老模型小鼠的抗氧化作用 [J], 刘春红;金钟斗;韩宝瑞2.参景固本方对D-半乳糖致亚急性衰老模型小鼠的抗氧化作用 [J], 周丽雅;李欣;柴晶美;杨阿龙3.金银花水煎剂对D-半乳糖致衰老模型小鼠的抗氧化作用 [J], 张莹莹;王银环;史亚;甘力;程东庆4.不同植物油脂对D-半乳糖衰老模型小鼠的抗氧化作用 [J], 杨晓君;商雪珂;买迪娜木·阿布力米提;海荣;丁泽人5.地黄饮子加减方对D-半乳糖致衰老模型小鼠抗氧化作用的影响 [J], 谢芳;谢宁因版权原因,仅展示原文概要,查看原文内容请购买。
土大黄根提取物对小鼠非特异性免疫功能的影响

土大黄根提取物对小鼠非特异性免疫功能的影响尤力都孜·买买提;阿布都吉力力·阿布都艾尼;热比姑丽·伊斯拉木【摘要】Objective To explore the effect of Rumex L. root extract on non-specific immune function of mice. Methods Carbon clear-ance test was used in mice(inert carbon granule clearance method)to observe the effect of Rumex L. extract administration(1,2, 4 g/kg)on mice in the general status,body weight,spleen and thymus index and then clearance index. Results There were no obvious abnormality in mice of Rumex L. root extract(1,2,4 g/kg)in the general status,body weight,spleen and thymus index( P > 0. 05);though the clearance index was increased in normal mice,there was no significant changes ( P > 0. 05). Conclusion Rumex L. root extract has a certain influence on non-specific immunefunction(phagocytosis of mononuclear scavenger system)of mice.%目的:探讨土大黄根提取物对正常小鼠非特异性免疫功能的影响。
卵巢切除大鼠降钙素基因相关肽、乙酰胆碱和去甲肾上腺素血管效应的变化
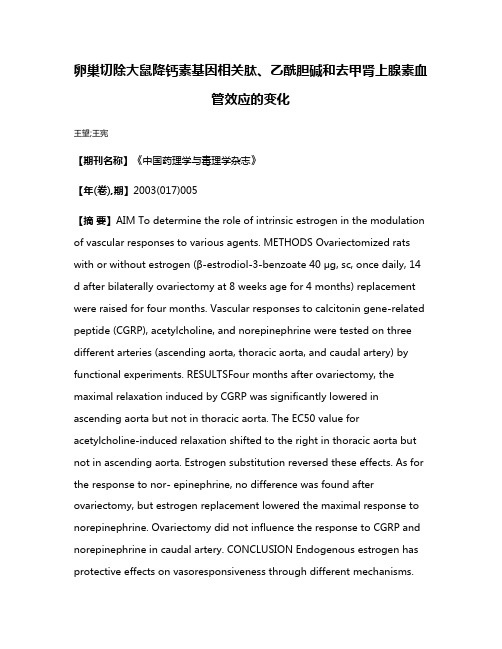
卵巢切除大鼠降钙素基因相关肽、乙酰胆碱和去甲肾上腺素血管效应的变化王望;王宪【期刊名称】《中国药理学与毒理学杂志》【年(卷),期】2003(017)005【摘要】AIM To determine the role of intrinsic estrogen in the modulation of vascular responses to various agents. METHODS Ovariectomized rats with or without estrogen (β-estrodiol-3-benzoate 40 μg, sc, once daily, 14 d after bilaterally ovariectomy at 8 weeks age for 4 months) replacement were raised for four months. Vascular responses to calcitonin gene-related peptide (CGRP), acetylcholine, and norepinephrine were tested on three different arteries (ascending aorta, thoracic aorta, and caudal artery) by functional experiments. RESULTSFour months after ovariectomy, the maximal relaxation induced by CGRP was significantly lowered in ascending aorta but not in thoracic aorta. The EC50 value for acetylcholine-induced relaxation shifted to the right in thoracic aorta but not in ascending aorta. Estrogen substitution reversed these effects. As for the response to nor- epinephrine, no difference was found after ovariectomy, but estrogen replacement lowered the maximal response to norepinephrine. Ovariectomy did not influence the response to CGRP and norepinephrine in caudal artery. CONCLUSION Endogenous estrogen has protective effects on vasoresponsiveness through different mechanisms.This study gives further support to the hormone replacement therapy for postmenopausal women.%目的研究雌性大鼠卵巢切除和雌激素替代治疗4个月后,血管活性物质对离体动脉作用的改变.方法采用双侧卵巢切除的雌性大鼠, 分为假手术组、去卵巢组(8周龄切除双侧卵巢)和雌激素替代组(苯甲雌二醇40 μg, sc,每日1次,卵巢切除后14 d开始给药至切除后4个月).于给药结束时取升主动脉、胸主动脉及尾动脉,进行离体血管功能实验,观察对降钙素基因相关肽(CGRP)、乙酰胆碱(ACh)及去甲肾上腺素(NE)的反应.结果卵巢切除4个月使CGRP引起的大鼠升主动脉舒张最大效应明显降低,而对胸主动脉无影响;同时ACh对胸主动脉的舒张效应曲线明显右移,而对升主动脉无影响.雌激素替代治疗使上述变化反转.去卵巢组的各类动脉对NE 的反应性无变化,而雌激素替代组的升主动脉和胸主动脉对NE引起的最大收缩效应明显降低.在尾动脉,去卵巢组和雌激素替代组的CGRP和NE反应性均无改变.结论内源性雌激素可以通过多种途径调节血管反应性,发挥其心血管保护作用.本研究进一步提示对绝经期妇女进行激素替代治疗的重要性.【总页数】7页(P338-344)【作者】王望;王宪【作者单位】北京大学第三医院血管医学研究所,北京,100083;北京大学第三医院血管医学研究所,北京,100083【正文语种】中文【中图分类】R972【相关文献】1.大鼠脊髓损伤后运动终板内乙酰胆碱酯酶和降钙素基因相关肽的变化 [J], 张皑峰;欧喜超;杨朝阳;刘玉军;鲁强;李晓光2.去甲肾上腺素及血管紧张素Ⅱ对大鼠心肌细胞胰岛素受体及胰岛素受体底物1酪氨酸磷酸化的综合效应 [J], 刘扬;李竹琴;张志刚3.大鼠腹腔注射荷包牡丹碱和阿托品对电针镇痛效应及其脑内去甲肾上腺素含量变化的影响 [J], 王友京;王双坤4.去甲肾上腺素在大鼠缰核引起的心血管效应及其机制 [J], 杨绍年;王绍;唐毓环5.一氧化氮对去甲肾上腺素在大鼠孤束核内引起的心血管效应的影响 [J], 欧阳明;葛光辉;王绍因版权原因,仅展示原文概要,查看原文内容请购买。
- 1、下载文档前请自行甄别文档内容的完整性,平台不提供额外的编辑、内容补充、找答案等附加服务。
- 2、"仅部分预览"的文档,不可在线预览部分如存在完整性等问题,可反馈申请退款(可完整预览的文档不适用该条件!)。
- 3、如文档侵犯您的权益,请联系客服反馈,我们会尽快为您处理(人工客服工作时间:9:00-18:30)。
Journal of Experimental Botany,Vol.62,No.1,pp.21–37,2011 doi:10.1093/jxb/erq281Advance Access publication20September,2010REVIEW PAPERRoot responses to cadmium in the rhizosphere:a reviewAlexander Lux1,*,Michal Martinka1,Marek Vaculı´k1and Philip J.White21Department of Plant Physiology,Faculty of Natural Sciences,Comenius University in Bratislava,Mlynska´dolina B2,84215Bratislava, Slovakia2Scottish Crop Research Institute,Invergowrie,Dundee DD25DA,UK*To whom correspondence should be addressed:E-mail:lux@fns.uniba.skReceived17June2010;Revised17August2010;Accepted18August2010AbstractThis article reviews the responses of plant roots to elevated rhizosphere cadmium(Cd)concentrations.Cadmium enters plants from the soil solution.It traverses the root through symplasmic or apoplasmic pathways before entering the xylem and being translocated to the shoot.Leaf Cd concentrations in excess of5–10m g g21dry matter are toxic to most plants,and plants have evolved mechanisms to limit Cd translocation to the shoot.Cadmium movement through the root symplasm is thought to be restricted by the production of phytochelatins and the sequestration of Cd-chelates in vacuoles.Apoplasmic movement of Cd to the xylem can be restricted by the development of the exodermis,endodermis,and other extracellular barriers.Increasing rhizosphere Cd concen-trations increase Cd accumulation in the plant,especially in the root.The presence of Cd in the rhizosphere inhibits root elongation and influences root anatomy.Cadmium concentrations are greater in the root apoplasm than in the root symplasm,and tissue Cd concentrations decrease from peripheral to inner root tissues.This article reviews current knowledge of the proteins involved in the transport of Cd across root cell membranes and its detoxification through sequestration in root vacuoles.It describes the development of apoplastic barriers to Cd movement to the xylem and highlights recent experiments indicating that their maturation is accelerated by high Cd concentrations in their immediate locality.It concludes that accelerated maturation of the endodermis in response to local Cd availability is of functional significance in protecting the shoot from excessive Cd loads.Key words:Accumulation,apoplasm,cadmium,endodermis,maize,root,suberin lamellae,symplasm,tissue asymmetry, transport.IntroductionCadmium(Cd)is toxic to plant cells,even at low concen-trations.Leaf concentrations greater than5–10l g Cd gÀ1 DM are toxic to most plants(White and Brown,2010), although some ecotypes of a few plant species have adapted to grow on soils with high Cd concentrations and can tolerate leaf concentrations in excess of100l g Cd gÀ1DM(Reeves and Baker,2000;Broadley et al.,2001;Verbruggen et al., 2009).These Cd-hyperaccumulator plants include ecotypes of Noccaea(formerly Thlaspi)caerulescens(J&C Presl.)FK Mey (Reeves et al.,2001),Arabidopsis(formerly Cardaminopsis) halleri(L.)O’Kane and Al-Shehbaz(Bert et al.,2002),Sedum alfredii Hance(Yang et al.,2004),Viola baoshanensis Shu, Liu et Lan.(Wei et al.,2004),Thlaspi praecox Wulf.(Vogel-Mikusˇet al.,2005),Picris divaricata Vant.(Tang et al.,2009),and Phytolacca americana L.(Liu et al.,2010b).The roots of some of these exceptional plant species proliferate in Cd-enriched patches of soil,which contrasts with the behaviour of roots of most plant species that generally avoid such patches(Whiting et al.,2000;Liu et al.,2010a).To prevent Cd accumulation in shoot tissues,plants have evolved various mechanisms to restrict the entry of Cd to the xylem.This article reviews our current knowledge of these mechanisms, which include(i)the production of Cd-chelates in the cytoplasm of root cells and the sequestration of Cd-chelates in the vacuole to restrict Cd delivery to the xylem from the symplast,and(ii)the development of physical barriers to the extracellular movement of Cd to the xylem to restrict Cd delivery to the xylem from the apoplasm.ªThe Author[2010].Published by Oxford University Press[on behalf of the Society for Experimental Biology].All rights reserved. For Permissions,please e-mail:journals.permissions@ at South China Agricultural University on December 5, Downloaded fromCadmium in the soilTo limit Cd concentrations in edible produce,Cd concen-trations lower than3l g gÀ1dry soil have been recommended for agriculture and horticulture(Mengel et al.,2001). Cadmium concentrations in non-polluted soils increase with clay concentration,but are generally lower than1l g gÀ1dry soil(Mengel et al.,2001).Cadmium is concentrated in the topsoil,where it is associated with organic matter.Solutions extracted from non-polluted soils generally have Cd concen-trations less than40–300nM(Wagner,1993).Cadmium availability to plants is greater in acid soils(Mengel et al., 2001;Tudoreanu and Phillips,2004;Kirkham,2006),and its solubility is increased by root exudates(Zhu et al.,1999). Cadmium occurs in the soil solution predominantly as Cd2+, but also as Cd-chelates(Tudoreanu and Phillips,2004).Low Cd2+concentrations in the soil solution,in combination with low diffusion coefficients for Cd2+in aqueous solutions, suggest that transpiration-driven mass-flow of the soil solution will dominate the delivery of Cd2+to plant roots (Sterckeman et al.,2004).This is consistent with reports that Cd accumulation by plants grown in soil is directly related to transpiration(Ingwersen and Streck,2005).Higher soil Cd concentrations can occur either naturally or through anthro-pogenic activities(Mengel et al.,2001;He et al.,2005; Kirkby and Johnson,2008).Natural mineral outcrops can be enriched in Cd through the weathering of Cd-rich rocks. Cadmium pollution of the environment has occurred through the mining and refining of metal ores,and through the appli-cation of Cd-containing phosphate fertilizers,sewage sludge, and municipal composts to agricultural soils.The ability to grow on soils with high Cd concentrations is generally related to the ability of roots to exclude Cd from the plant and/or of plant tissues to chelate Cd as a non-toxic com-pound or sequester it in a non-vital cellular compartment. Plants tolerating high Cd concentrations,and especially plants accumulating this metal in their above-ground parts, have potential utility for the phytostabilization and phytor-emediation of contaminated soils(Raskin and Ensley,2000; Schwitzgue´bel et al.,2009).Cadmium uptake by plant rootsIn nature,shoot Cd concentrations vary greatly.Although much of this variation can be attributed to environmental factors,there is appreciable phylogenetic variation in shoot Cd concentrations(Broadley et al.,2001;Watanabe et al., 2007).When grown in the same environment,shoot Cd concentrations are generally highest in species from the Caryophyllales and Lamiales,and lowest in monocot species (Broadley et al.,2001).Shoot Cd concentrations are de-termined largely by Cd entry to the root,sequestration within root vacuoles,translocation in the xylem and phloem, and dilution within the shoot through growth.Cadmium concentrations are often(but not always)greater in roots than in shoots,suggesting that Cd transport to the xylem is restricted in most plants,and lowest in seeds,fruits,and tubers,suggesting that Cd is not readily translocated in the phloem(Seregin and Kozhevnikova,2008;Conn and Gilliham,2010).Grafting experiments have suggested that shoot Cd concentrations in Nicotiana(N.tabacum L.and N.rustica L.;Wagner et al.,1988),Solanum(S.integrifolium, S.melongena,and S.torvum;Arao et al.,2008),and Noccaea/ Thlaspi(Noccaeae caerulescens and Thlaspi perfoliatum; Guimara˜es et al.,2009)are controlled by root properties. Shoot Cd concentration often increases to a maximum value with increasing Cd concentration in the soil(Logan et al.,1997;Zhao et al.,2003;Sterckeman et al.,2004;Vogel-Mikusˇet al.,2005;Chen et al.,2008;Peng et al.,2009;Liu et al.,2010b),bulk soil solution,or nutrient solution(Yang et al.,1995;Perriguey et al.,2008;Street et al.,2009),al-though non-saturating relationships have also been reported (Salt et al.,1995;Ingwersen and Streck,2005).The con-centration dependence of Cd uptake from hydroponic solu-tions measured over short periods into either excised roots or intact plants generally follows the sum of a single Michaelis–Menten component plus a linear component(Table1).The linear component is often attributed to tight Cd binding to cell walls,but it could also represent an apoplasmic Cdflux to the xylem(cf.White,2001;White et al.,2002;Broadley et al.,2007).Estimates of the K m value for‘high-affinity’Cd uptake commonly fall between20–1000nM(Table1). Cadmium uptake and accumulation by plant roots is generally inhibited by La3+,Ca2+,Cu2+,Fe2+,Zn2+or Mn2+ in the rhizosphere solution(Cataldo et al.,1983;Costa and Morel,1993,1994;Cohen et al.,1998;Lombi et al.,2001; Hart et al.,2002;Zhao et al.,2002;Berkelaar and Hale, 2003a;Han et al.,2006;Zhao et al.,2006),but Cd uptake by the Cd-hyperaccumulating Ganges ecotype of N.caerulescens was not inhibited by divalent cations nor by La3+(Lombi et al.,2001;Zhao et al.,2002).The presence of organic acid anions in hydroponic solutions increases the capacity of both the Michaelis–Menten component and the linear component of Cd uptake,but the reasons for this are unclear(Berkelaar and Hale,2003a;Han et al.,2006).Cadmium can enter root cells as Cd2+through ZIP(Zinc-regulated transporter/Iron-regulated transporter-like Protein) transporters,such as orthologues of AtIRT1and TcZNT1/ TcZIP4,through orthologues of the wheat TaLCT1transpor-ter,or via cation channels,such as depolarization-activated calcium channels(DACC),hyperpolarization activated cal-cium channels(HACC),and voltage-insensitive cation chan-nels(VICC),all of which are relatively non-selective between cations(Fig.1;Clemens et al.,1998;Cohen et al.,1998; White and Broadley,2003;White,2005;Plaza et al.,2007; DalCorso et al.,2008;Pedas et al.,2008;Verbruggen et al., 2009).In addition,Cd might enter root cells as Cd-chelates through YSL(Yellow-Stripe1-Like)proteins(Curie et al., 2009).Cadmium can then reach the stele through a symplas-mic pathway formed by the cytoplasms of individual root cells connected by plasmodesmata.The Cd species trans-ported through the symplasm are unknown,but could include Cd2+or Cd-chelates(Verbruggen et al.,2009). Cadmium is loaded from the symplasm into the xylem by heavy metal P1B-ATPases,such as orthologues of AtHMA2and AtHMA4,and possibly also by YSL proteins22|Lux et al.at South China Agricultural University on December 5, Downloaded from(Mills et al.,2005;DalCorso et al.,2008;Wong and Cobbett,2008;Verbruggen et al.,2009).The Arabidopsis ABC trans-porter AtPDR8has been implicated in Cd efflux across the plasma membrane of root hairs and epidermal cells (Kim et al.,2007).In many plant species,Cd tolerance is related to Cd accumulation in the vacuole (Chardonnes et al.,1998;Cosio et al.,2005;Korenkov et al.,2007;Seregin and Kozhevnikova,2008).Cadmium can be translocated across the tonoplast by H +/Cd 2+-antiporters,such as orthologues of AtCAX2and AtCAX4(Korenkov et al.,2007,2009),by heavy metal P 1B -ATPases,such as orthologues of AtHMA3(Morel et al.,2009),and as Cd-chelates by ABC transporters,such as orthologues of AtMRP3(Tommasini et al.,1998;Table 1.Published relationships between cadmium (Cd)uptake (V )by excised roots or intact plants and Cd in the external solution ([Cd]ext ),described by Michaelis–Menten functions using the terms V max (V ,when [Cd]ext ¼N )and K m ([Cd]ext ,when V ¼0.5V max ),and a linear term k (V /[Cd]ext )*Original data expressed on the basis of root surface area,V max ¼400pmol m À2s À1.**Values converted from DM to FW values using the conversion factor DM/FW ¼0.1.[Cd]ext (m M)K m (nM)V max(nmol g À1FW h À1)K m (m M)V max(nmol g À1FW h À1)k(nmol g À1FW h À1m M À1)ReferenceSoybean (Glycine max (L.)Merr.)Intact plants 0.000002.5–0.57622.9 1.2232ND Cataldo et al.,1983Maize (Zea mays L.)Intact plants 0.0063–0.16430–100*NDNDND Mullins andSommers,1986Maize (Zea mays L.)Intact plants 0.25–5.020020.31ND ND 16Han et al.,2006Maize (Zea mays L.)Intact plants 0.00001–100ND ND ND ND Yes Perriguey et al.,2008Maize (Zea mays L.)Excised roots 0.05–5026023.6**ND ND 3.6**Redjala et al.,2009Lupin (Lupinus albus L.)Intact plants 0.00005–5.04211.6NDNDND Costa and Morel,1993Lettuce (Lactuca sativa L.)Intact plants 0.00005–5.08–3032–43**0.011–0.0542420–7740**ND Costa and Morel,1994Pea (Pisum sativum L.)[Fe-replete]Excised roots 1–10060034ND ND 5.3Cohen et al.,1998Bread wheat(Triticum astivum L.)Intact plants 0.05–1.252026ND ND 28Hart et al.,1998Bread wheat(Triticum astivum L.)Intact plants 0.05–1.55933ND ND Yes Hart et al.,2002Durum wheat (Triticum turgidum L.var.durum )Intact plants0.05–1.254029NDND22Hart et al.,1998Durum wheat (Triticum turgidum L.var.durum )Intact plants 0.05–1.56738ND ND Yes Hart et al.,2002Durum wheat (Triticum turgidum L.var.durum )Intact plants 0.005–1.81660.87ND ND 1.69Harris and Taylor,2004Noccaeacaerulescens (Prayon)Intact plants 0.2–5026033ND ND 6.0Lombi et al.,2001Noccaeacaerulescens (Prayon)Intact plants 0.2–5093021.8ND ND 4.2Lombi et al.,2002Noccaeacaerulescens (Ganges)Intact plants 0.2–50180160ND ND 11.2Lombi et al.,2001Noccaeacaerulescens (Ganges)Intact plants 0.2–501000187.6ND ND 3.6Lombi et al.,2002Noccaeacaerulescens (Ganges)Intact plants <5450143ND ND ND Zhao et al.,2002Noccaeacaerulescens (Nc-H)Excised roots 0.05–50390130**ND ND 1.38**Redjala et al.,2009Noccaeacaerulescens (Nc-L)Excised roots 0.05–507023.4**ND ND 2.22**Redjala et al.,2009Arabidopsis halleri Intact plants 0.2–1035039.7ND ND 15Zhao et al.,2006Rice (Oryza sativa L.)Intact plants 0.25–50254044ND ND 0.8He et al.,2007Eggplant(Solanum melongena )Intact plants 0.04–1.2380270**ND ND ND Mori et al.,2009Solanum torvumIntact plants0.04–1.2353**NDNDNDMori et al.,2009Root responses to cadmium |23at South China Agricultural University on December 5, 2010 Downloaded fromCobbett,2000;DalCorso et al.,2008;Verbruggen et al.,2009).Within plant cells,Cd is bound to S-containing ligands,such as those present in glutathione,metallothio-neins,and phytochelatins (DalCorso et al.,2008).In many plant species,the synthesis of phytochelatins is induced by Cd exposure (see Salt et al.,1995;Schat et al.,2002;Clemens,2006;Ernst et al.,2008;Seregin and Kozhevnikova,2008;Persson et al.,2006)and Cd is often sequestered in the vacuole as Cd-phytochelatin complexes (Cobbett,2000;Clemens,2006;Ernst et al.,2008).The involvement of phytochelatins in Cd detoxification is consistent with observations that mutants deficient in phytochelatin synthesis show lower Cd tolerance than wild-type plants and mutants with enhanced phytochelatin synthesis can show higher Cd tolerance than wild-type plants (Cobbett,2000;Clemens,2006).However,differ-ences in Cd tolerance among natural ecotypes of several plant species appear to be unrelated to differences in phytochelatin synthesis,suggesting additional mechanisms of Cd-detoxification (Schat et al.,2002).In some plant species,metallothioneins play an important role in Cd detoxification (Ernst et al.,2008;Shim et al.,2009).The production of metallothioneins is also induced by exposure to Cd,and plants genetically engineered to produce metal-lotheioneins in greater concentrations show increased Cd tolerance (see DalCorso et al.,2008;Korenkov et al.,2009).Cadmium is thought to be exported from the vacuole by NRAMP (Natural Resistance-Associated Macrophage Pro-tein)transporters,such as orthologues of AtNRAMP3and AtNRAMP4(Thomine et al.,2003;Verbruggen et al.,2009).It is also possible for Cd 2+and Cd-chelates to reach the xylem solely via an extracellular,apoplasmic pathway in regions of the root lacking a Casparian band (Berkelaar and Hale,2003b ;Lux et al.,2004).The uptake of cationic ele-ments reaching the xylem via an apoplasmic pathway is generally restricted to the extreme root tip and to regions in which lateral roots are being initiated (White,2001;Moore et al.,2002).Although the relative contributions of the sym-plastic and apoplasmic pathways to the delivery of Cd to the xylem are unknown,it is likely that the relative contri-bution of the apoplasmic pathway will increase as the Cd concentration in the rhizosphere solution increases,as has been proposed for both Zn and Na (White et al.,2002;Plett and Møller,2010).Supporting the presence of an apoplasmic pathway,are observations that the root tip is the most active region of the root for Cd 2+influx (Pin ˜eros et al.,1998),and that Cd accumulation by wheat cultivars is positively correlated with their number of root apices (Berkelaar and Hale,2000).A linear component to the concentration dependence of Cd uptake on Cd concentration in the nutrient solution is almost universally observed in short duration studies on hydroponically-grown plants (Table 1).This phenomenon can be interpreted as the result of an apoplasmic Cd flux to the xylem.In challenging this view,Xing et al.(2008)showed that,among accessions of N.caerulescens with contrasting abilities to take up Cd and Zn,the amount of Cd taken up by roots over a 24h period and translocated to the shoot was inversely related to apoplasmic water flows assayed using a membrane-impermeable fluorescentdye.Fig.1.Proteins thought to be responsible for Cd influx to the symplasm,sequestration in the vacuoles of root cells,and efflux to the rhizosphere and to the xylem (see text for details).24|Lux et al.at South China Agricultural University on December 5, 2010 Downloaded fromHowever,this is inconsistent with studies of the effects of transpiration on Cd accumulation by other plant species (Ingwersen and Streck,2005).Reducing transpiration by the addition of ABA to the nutrient solution has also been shown to reduce both xylem sap Cd concentration and shoot Cd accumulation of hydroponically grown plants (Salt et al.,1995;Hsu and Kao,2003;Zhao et al.,2006;Liu et al.,2010b),but this has been interpreted as an effect of ABA on symplastic loading of Cd into the xylem since Cd uptake by roots themselves was unaffected by the presence of ABA(Salt et al.,1995;Zhao et al.,2006).More persua-sive arguments for symplasmic delivery of Cd to the xylem are(i)that there is competition between Cd2+and other cations for uptake by roots(Hart et al.,2002)and(ii)the contrasting abilities of N.caerulescens accessions to accu-mulate Cd and Zn independently in the shoot provides evidence of transport selectivity(Xing et al.,2008),both of which can be taken as evidence for protein-mediated symplasmic transport(White,2001).Barriers to apoplasmic movement of solutes to the xylemThe isolation of the stele from the peripheral cell layers of the root is critical for the control of solute transport to the shoot.It is effected by cell wall impregnations and the most important impregnating substance in this context is suberin (Franke and Schreiber,2007).Cadmium uptake by plant roots is generally restricted to young subapical regions of actively growing roots(Pin˜eros et al.,1998).The peripheral cell layers in these regions are specialized for solute uptake.The epidermal layer,which constitutes the outermost cell layer and differs from the epidermal layer of above-ground plant parts both in lacking cutin and through the development of root hairs,is termed the rhizodermis(von Guttenberg,1968).The rest of the peripheral tissues are termed the cortex.The endodermis separates the cortex from the stele and extracellular move-ment of solutes to the xylem is restricted by suberin deposi-ted in endodermal cell walls.Suberin,together with lignins, form the impregnation material of Casparian bands de-veloped in radial and transverse endodermal cell walls (Schreiber et al.,1999;White,2001).This impregnation,the lack of intercellular spaces between endodermal cells,and a tight junction between cell walls and the plasma mem-brane form the apoplasmic barrier of the root(White, 2001).The endodermis with its Casparian bands represents a considerable,but not impenetrable,barrier to solute movement through the apoplasm(Steudle et al.,1993; White,2001;Ranathunge et al.,2005).Casparian bands represent thefirst endodermal ontogenic stage(Stage I),which is only rarely(e.g.in some aquatic species;Seago,2002)thefinal stage.The deposition of lamellar suberin,in this case on the whole inner cell wall surface,is the commonly occurring second endodermal ontogenic stage(White,2001).Stage II endodermis presents a more complete apoplasmic barrier to the radialflow of water and solutes to the xylem in more mature parts of theroot(Melchior and Steudle,1993;Peterson et al.,1993; Steudle and Peterson,1998;White,2001).Nevertheless,the absolute amount of suberin deposited in root cell walls affects the radial transport of water and ions,as demon-strated by the enhanced suberin1(esb1)mutant of Arabidop-sis thaliana Heynh.(Baxter et al.,2009).This mutant has elevated amounts of suberin in the root,most likely in the endodermis,reduced waterflow to the xylem,and a decreasein shoot Ca,Mn,and Zn accumulation(Baxter et al.,2009). However,quantitative differences in root suberin concen-trations may not be the only factor influencing the apo-plasmic movement of water and solutes to the xylem,andboth the chemical nature of the suberins,together with the microstructure of deposits,must also be taken into consid-eration(Schreiber et al.,2005).Differences in suberin composition along the root axis during the development ofthe endodermis are indicated by an increase in fatty acidx-hydroxylation(Thomas et al.,2007;Ho¨fer et al.,2008). However,association of these changes with functional properties of suberized barriers in the endodermis remainsto be demonstrated.In some plant species,more distant from the root apex,ineven older parts of the root,the endodermis may pass to thethird stage(von Guttenberg,1968;White,2001).Stage III endodermis is characterized by thick cellulose secondarywalls(sometimes classified as tertiary walls)deposited overthe suberin lamellae.This cell wall layer,together with the original primary wall,is often lignified,and in some species impregnation of walls with silicon may occur(Sangster and Parry,1976;Lux et al.,1999).In Stage III,the function ofthe endodermis is already mostly mechanical and radial transport of water and solutes is limited(Melchior and Steudle,1993;White,2001).The gradual changes in cell wall composition of the endodermis can extend for a considerable distance along the root.Thefirst stage,characterized by Casparian bands,often starts very close to the root apex.The zone of gradual development of the second stage,the deposition of suberin lamellae,is usually very long.It may vary from several millimetres to several hundred millimetres from the root apex.Throughout this distance the number of endodermalcells without suberin lamellae decreases,and the cells not covered by suberin lamellae are called passage cells.Passagecells can be present even when the majority of endodermalcells have entered the third stage of development.Indeed, passage cells can remain in thefirst state permanently, although in the majority of the plant species this does not occur.Hypodermal layers,characterized by a gradual thickeningof cell walls,are often present in older roots,especially in monocotyledons.A suberized periderm is developed in olderroots of dicotyledons and gymnosperms.Periderm has been shown to act as a barrier preventing the movement of waterand ions(Vogt et al.,1983),gases(De Simone et al.,2003),and pathogen incursion(Lulai and Corsini,1998).From a functional viewpoint,these older regions of the root con-tribute little to water uptake(Melchior and Steudle,1993)Root responses to cadmium|25at South China Agricultural University on December 5, Downloaded fromand are mostly engaged in the long-distance transport of water and solutes.This function is concentrated in inner root tissues,within the vascular cylinder,or in the secondary vascular tissues.In the majority of angiosperms another apoplasmic barrier,the exodermis,can develop in parallel with the endodermis(Perumala et al.,1990;Peterson and Perumala, 1990;Hose et al.,2001;Ma and Peterson,2003).The exodermis develops in the same three stages as the endo-dermis.The exodermis can be uniseriate or multiseriate,in contrast to the uniseriate endodermis.The exodermis usually develops at a greater distance from the root apex than the endodermis(Ma and Peterson,2003).However, environmental conditions can modify the rate of develop-ment of the exodermis(Zimmermann and Steudle,1998) and accelerated development of the exodermis has been associated with reduced Cd uptake by roots(T Redjala and I Zelko,personal communication).In some plant species, and under specific environmental conditions,the exodermis may differentiate earlier than the endodermis.This has been observed in some wetland plants(Seago et al.,1999;Soukup et al.,2002),in tea(Homma et al.,2000;Tanimoto et al., 2004),and in maize(Zea mays L.)grown in soil(T Redjala and I Zelko,personal communication).The importance of the exodermis as an environmentally variable barrier to the uptake of water and ions was recognized by Peterson et al. (1993),and several subsequent studies have confirmed this conclusion(Peterson,1997;Meyer et al.,2009).Under some conditions,such as in hydroponics,the exodermis can be absent,even in species in which it is normally present (Zimmerman and Steudle,1998).The rhizodermis,exodermis,and endodermis have all been shown to act as barriers to the apoplasmic movement of toxic elements,including Cd(Gierth et al.,1999;White, 2001;Enstone et al.,2003;Seregin et al.,2004;Seregin and Kozhevnikova,2008).The additional peri-endodermal layer of cells with lignified cell walls present in N.caerulescens may function similarly(Zelko et al.,2008).These apoplas-mic barriers develop closer to the root apex when roots are exposed to high concentrations of potentially toxic ele-ments.Accelerated development of both the endodermis and exodermis have been observed in various plant species in response to salinity(Reinhardt and Rost,1995;Karahara et al.,2004),and the multiple environmental stresses caused by cultivation in municipal solid waste slag with high salt and heavy metal content have been shown to induce extensive thickening of the inner tangential walls of maize endodermal cells(Degenhardt and Gimmler,2000).Exposure to Cd has been found to result in the formation of Casparian bands and suberin lamellae closer to the root apex in several plant species,including A.thaliana (Schreiber et al.,1999),Silene dioica(Martinka and Lux, 2004),woody shrub species such as Karwinskia humboldti-ana(Zelko and Lux,2004),and maize(Fig.2;Vaculı´k et al., 2009).Maturation of the endodermis closer to the root apex can be attributed partly to a reduction in the rate ofrootFig.2.The development of endodermal suberin lamellae in the roots of maize plants after10d growth in Hoagland’s solution containingno cadmium(Cd0)or5l M Cd(NO3)2.4H2O(Cd5).Three regions of the root can be distinguished:A,a region in which endodermalsuberin lamellae are fully developed(solid green lines),B,a region in which the suberin lamellae are partially developed(broken green lines),and C,a region lacking endodermal suberin lamellae.Because the length of roots grown in the absence and presence of Cddiffered,the distance from the root tip is expressed as percentage of the total root length.Endodermal suberin lamellae developed closerto the root apex in roots exposed to Cd,when expressed in either absolute or percentage terms.White arrows indicate suberin lamellaein the endodermis.26|Lux et al.at South China Agricultural University on December 5, Downloaded from。