Biohydrogen production by anaerobic fermentation
生物制氢 发酵工艺流程
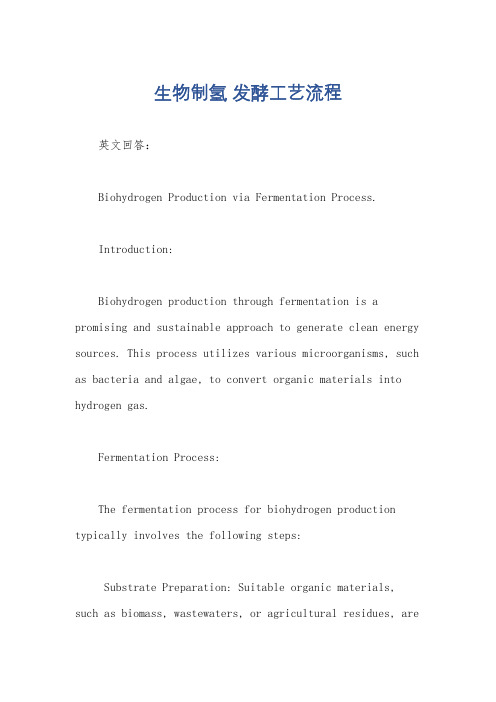
生物制氢发酵工艺流程英文回答:Biohydrogen Production via Fermentation Process.Introduction:Biohydrogen production through fermentation is a promising and sustainable approach to generate clean energy sources. This process utilizes various microorganisms, such as bacteria and algae, to convert organic materials into hydrogen gas.Fermentation Process:The fermentation process for biohydrogen production typically involves the following steps:Substrate Preparation: Suitable organic materials, such as biomass, wastewaters, or agricultural residues, arepre-processed to optimize their biodegradability.Fermentation: The substrate is fermented in an anaerobic environment, typically using a mixed culture of microorganisms or specific bacterial strains. During this stage, the organic matter is converted into intermediate compounds, including volatile fatty acids (VFAs).Hydrogen Production: Specialized microorganisms, such as hydrogen-producing bacteria, utilize the VFAs as substrates to produce hydrogen gas through anaerobic fermentation.Gas Separation: The produced hydrogen gas is separated from other fermentation gases, such as methane and carbon dioxide, using techniques like membrane filtration or pressure swing adsorption (PSA).Advantages of Fermentation Process:Renewable and Sustainable: Uses organic waste materials as substrates, reducing greenhouse gas emissionsand promoting waste management.High Hydrogen Yield: Can achieve relatively high hydrogen yields compared to other bio-production methods.Cost-Effective: Fermentation processes can be more economical than other hydrogen production technologies, especially when utilizing inexpensive biomass resources.Environmentally Friendly: Produces hydrogen gas as a clean energy source, reducing air pollution and promoting carbon neutrality.Challenges and Considerations:Substrate Availability: Securing a reliable and sustainable supply of organic substrates can be challenging.Process Efficiency: Optimizing fermentation conditions, such as pH, temperature, and inoculum composition, iscrucial for maximizing hydrogen production efficiency.Hydrogen Purification: Removing impurities and separating hydrogen from other fermentation gases can be energy-intensive and require advanced separation technologies.Long-Term Stability: Maintaining stable microbial communities and fermentation conditions over prolonged periods can be challenging.Future Research Directions:Ongoing research efforts focus on improving the fermentation process for biohydrogen production. Key areas include:Substrate Optimization: Identifying and pretreating suitable biomass feedstocks to enhance hydrogen yields.Microbial Engineering: Developing genetically modified microorganisms with enhanced hydrogen production capabilities.Process Integration: Exploring innovative approaches to combine fermentation with other technologies, such as dark fermentation and photofermentation.Scale-Up and Commercialization: Demonstrating the feasibility and economic viability of large-scale biohydrogen production systems.中文回答:生物制氢发酵工艺流程。
乙酰半胱氨酸生产工艺流程
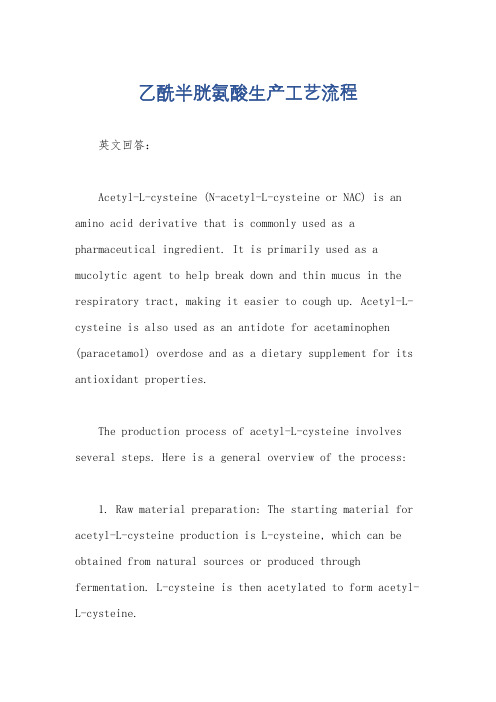
乙酰半胱氨酸生产工艺流程英文回答:Acetyl-L-cysteine (N-acetyl-L-cysteine or NAC) is an amino acid derivative that is commonly used as a pharmaceutical ingredient. It is primarily used as a mucolytic agent to help break down and thin mucus in the respiratory tract, making it easier to cough up. Acetyl-L-cysteine is also used as an antidote for acetaminophen (paracetamol) overdose and as a dietary supplement for its antioxidant properties.The production process of acetyl-L-cysteine involves several steps. Here is a general overview of the process:1. Raw material preparation: The starting material for acetyl-L-cysteine production is L-cysteine, which can be obtained from natural sources or produced through fermentation. L-cysteine is then acetylated to form acetyl-L-cysteine.2. Acetylation reaction: L-cysteine is reacted with acetic anhydride or acetic acid in the presence of a catalyst, such as sulfuric acid or hydrochloric acid. The reaction takes place under controlled conditions, typically at a specific temperature and pressure, to ensure highyield and purity of the product.3. Filtration and purification: After the acetylation reaction, the mixture is filtered to remove any solid impurities. The filtrate is then subjected to further purification steps, such as solvent extraction or chromatography, to remove any remaining impurities and obtain a highly pure acetyl-L-cysteine product.4. Drying and formulation: The purified acetyl-L-cysteine is dried to remove any residual moisture. It is then formulated into various dosage forms, such as tablets, capsules, or powders, depending on the intended use.5. Quality control: Throughout the production process, various quality control tests are conducted to ensure thepurity, potency, and safety of the acetyl-L-cysteine product. These tests may include assays for activeingredient content, impurity analysis, and microbiological testing.中文回答:乙酰半胱氨酸(N-乙酰半胱氨酸或NAC)是一种常用的药物成分,它是一种氨基酸衍生物。
白桦茸提取物多糖的分子式
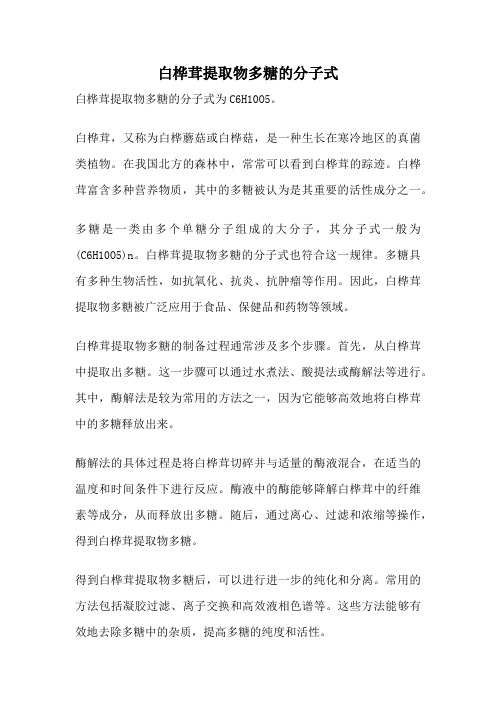
白桦茸提取物多糖的分子式白桦茸提取物多糖的分子式为C6H10O5。
白桦茸,又称为白桦蘑菇或白桦菇,是一种生长在寒冷地区的真菌类植物。
在我国北方的森林中,常常可以看到白桦茸的踪迹。
白桦茸富含多种营养物质,其中的多糖被认为是其重要的活性成分之一。
多糖是一类由多个单糖分子组成的大分子,其分子式一般为(C6H10O5)n。
白桦茸提取物多糖的分子式也符合这一规律。
多糖具有多种生物活性,如抗氧化、抗炎、抗肿瘤等作用。
因此,白桦茸提取物多糖被广泛应用于食品、保健品和药物等领域。
白桦茸提取物多糖的制备过程通常涉及多个步骤。
首先,从白桦茸中提取出多糖。
这一步骤可以通过水煮法、酸提法或酶解法等进行。
其中,酶解法是较为常用的方法之一,因为它能够高效地将白桦茸中的多糖释放出来。
酶解法的具体过程是将白桦茸切碎并与适量的酶液混合,在适当的温度和时间条件下进行反应。
酶液中的酶能够降解白桦茸中的纤维素等成分,从而释放出多糖。
随后,通过离心、过滤和浓缩等操作,得到白桦茸提取物多糖。
得到白桦茸提取物多糖后,可以进行进一步的纯化和分离。
常用的方法包括凝胶过滤、离子交换和高效液相色谱等。
这些方法能够有效地去除多糖中的杂质,提高多糖的纯度和活性。
白桦茸提取物多糖具有多种生物活性。
研究表明,它具有较强的抗氧化作用,能够清除体内的自由基,减少氧化损伤。
此外,白桦茸提取物多糖还具有抗炎、抗菌和免疫调节等作用,对于预防和治疗多种疾病具有潜在的应用前景。
白桦茸提取物多糖还可以应用于食品和保健品工业中。
由于其具有多种生物活性,可以作为功能性食品的添加剂,增强食品的营养价值和保健效果。
此外,白桦茸提取物多糖还可以制备成口服液、胶囊和片剂等形式的保健品,供人们日常补充营养和改善健康。
白桦茸提取物多糖是一种具有多种生物活性的活性成分。
其分子式为C6H10O5,符合多糖的一般特征。
经过提取、纯化和分离等工艺,可以得到高纯度的白桦茸提取物多糖,具有广泛的应用前景。
发酵法生产1,3-丙二醇的研究进展
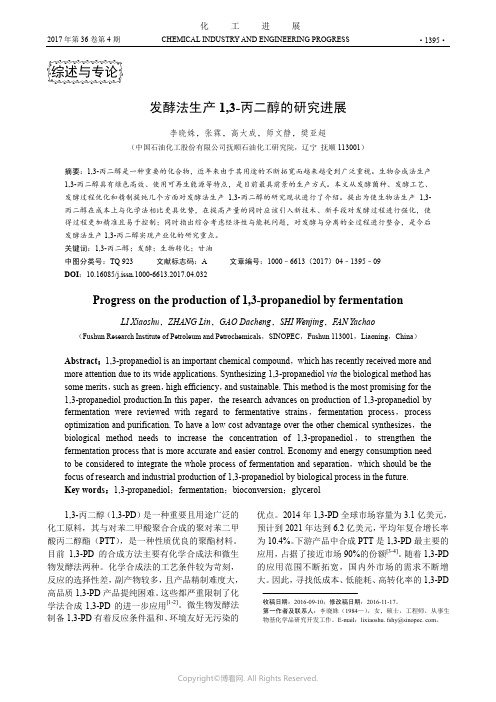
2017年第36卷第4期 CHEMICAL INDUSTRY AND ENGINEERING PROGRESS·1395·化 工 进展发酵法生产1,3-丙二醇的研究进展李晓姝,张霖,高大成,师文静,樊亚超(中国石油化工股份有限公司抚顺石油化工研究院,辽宁 抚顺113001)摘要:1,3-丙二醇是一种重要的化合物,近年来由于其用途的不断拓宽而越来越受到广泛重视。
生物合成法生产1,3-丙二醇具有绿色高效、使用可再生能源等特点,是目前最具前景的生产方式。
本文从发酵菌种、发酵工艺、发酵过程优化和精制提纯几个方面对发酵法生产1,3-丙二醇的研究现状进行了介绍。
提出为使生物法生产1,3-丙二醇在成本上与化学法相比更具优势,在提高产量的同时应该引入新技术、新手段对发酵过程进行强化,使得过程更加精准且易于控制;同时指出综合考虑经济性与能耗问题,对发酵与分离的全过程进行整合,是今后发酵法生产1,3-丙二醇实现产业化的研究重点。
关键词:1,3-丙二醇;发酵;生物转化;甘油中图分类号:TQ 923 文献标志码:A 文章编号:1000–6613(2017)04–1395–09 DOI :10.16085/j.issn.1000-6613.2017.04.032Progress on the production of 1,3-propanediol by fermentationLI Xiaoshu ,ZHANG Lin ,GAO Dacheng ,SHI Wenjing ,F AN Yachao(Fushun Research Institute of Petroleum and Petrochemicals ,SINOPEC ,Fushun 113001,Liaoning ,China )Abstract :1,3-propanediol is an important chemical compound ,which has recently received more andmore attention due to its wide applications. Synthesizing 1,3-propanediol via the biological method has some merits ,such as green ,high efficiency ,and sustainable. This method is the most promising for the 1,3-propanediol production.In this paper ,the research advances on production of 1,3-propanediol by fermentation were reviewed with regard to fermentative strains ,fermentation process ,process optimization and purification. To have a low cost advantage over the other chemical synthesizes ,the biological method needs to increase the concentration of 1,3-propanediol ,to strengthen the fermentation process that is more accurate and easier control. Economy and energy consumption need to be considered to integrate the whole process of fermentation and separation ,which should be the focus of research and industrial production of 1,3-propanediol by biological process in the future. Key words :1,3-propanediol ;fermentation ;bioconversion ;glycerol1,3-丙二醇(1,3-PD )是一种重要且用途广泛的化工原料,其与对苯二甲酸聚合合成的聚对苯二甲酸丙二醇酯(PTT ),是一种性质优良的聚酯材料。
STERIS Vaporized Hydrogen Peroxide (VHP) 生物消毒系统及服务
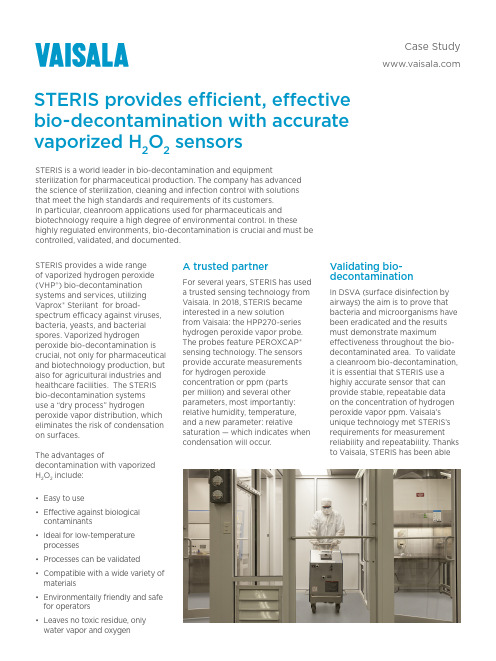
STERIS provides a wide range of vaporized hydrogen peroxide (VHP®) bio-decontamination systems and services, utilizing Vaprox® Sterilant for broad-spectrum efficacy against viruses, bacteria, yeasts, and bacterial spores. Vaporized hydrogenperoxide bio-decontamination is crucial, not only for pharmaceutical and biotechnology production, but also for agricultural industries and healthcare facilities. The STERIS bio-decontamination systems use a “dry process” hydrogenperoxide vapor distribution, which eliminates the risk of condensation on surfaces.The advantages ofdecontamination with vaporized H 2O 2 include:• Easy to use• Effective against biological contaminants• Ideal for low-temperature processes• Processes can be validated • Compatible with a wide variety of materials• Environmentally friendly and safe for operators• Leaves no toxic residue, only water vapor and oxygenA trusted partnerFor several years, STERIS has used a trusted sensing technology from Vaisala. In 2018, STERIS became interested in a new solutionfrom Vaisala: the HPP270-series hydrogen peroxide vapor probe. The probes feature PEROXCAP® sensing technology. The sensors provide accurate measurements for hydrogen peroxideconcentration or ppm (parts per million) and several other parameters, most importantly: relative humidity, temperature, and a new parameter: relativesaturation — which indicates when condensation will occur.Validating bio-decontaminationIn DSVA (surface disinfection by airways) the aim is to prove that bacteria and microorganisms have been eradicated and the results must demonstrate maximumeffectiveness throughout the bio-decontaminated area. To validate a cleanroom bio-decontamination, it is essential that STERIS use a highly accurate sensor that can provide stable, repeatable data on the concentration of hydrogen peroxide vapor ppm. Vaisala’s unique technology met STERIS’s requirements for measurement reliability and repeatability. Thanks to Vaisala, STERIS has been ableSTERIS provides efficient, effective bio-decontamination with accurate vaporized H 2O 2 sensorsSTERIS is a world leader in bio-decontamination and equipmentsterilization for pharmaceutical production. The company has advanced the science of sterilization, cleaning and infection control with solutions that meet the high standards and requirements of its customers. In particular, cleanroom applications used for pharmaceuticals andbiotechnology require a high degree of environmental control. In thesehighly regulated environments, bio-decontamination is crucial and must be controlled, validated, and documented. Case Studyto prove maximum effectiveness of bio-decontamination in clean rooms.“Today, Vaisala is the best technology on the market tomeasure the concentration of H 2O 2 reliably, accurately, and repeatably over time,” says Philippe Muylaert, Room Decontamination Service Specialist with STERIS SAS. “The Vaisala model HPP272 is the most effective sensor for bio-decontamination with hydrogen peroxide. Thus, we haveconfidence in the data, and we can prove to our customers the effectiveness of bio-decontamination cycles.”Muylaert appreciates that the HPP270 probes provide H 2O 2 concentration measurement curves throughout the bio-decontamination process in addition to real-time monitoring data.In-line data throughout a process is valuable for cycle development, especially to help determine pressure binary mixture, water concentration, temperature, etc.In-line measurement of vaporized H 2O 2To remain in a gaseous state,hydrogen peroxide vapor requires controlled parameters, including temperature, relative humidity, pressure and volume. Anydeparture from ideal conditions can cause hydrogen peroxide vapor to condense, essentially returning the H 2O 2 to its natural state: liquid. For STERIS’s dry method, it is necessary to avoid condensation that can lead to equipment deterioration.In the absence of hydrogen peroxide vapor, the relativehumidity of the air is equal to the relative saturation (1). When vaporized H 2O 2 is introduced, the relative saturation is greater than the relative humidity (2).During H 2O 2 vapor bio-decontamination processes, there is always water vapor in addition to hydrogen peroxide vapor. To control condensation, you need to know both the humidity of the air caused by water vapor and by hydrogen peroxide vapor.Relative saturation, which indicates the concentration of vaporizedhydrogen peroxide and water vapor in the air, is the only value thatrepresents both vapors. Monitoring the relative saturation value during a process is therefore crucial, because it indicates saturation point of the combined vapors: water and hydrogen peroxide.Reliable measurements mean reliable processesSTERIS systems required a probe capable of providing accurate measurements for hydrogen peroxide ppm, temperature, relative humidity and relative saturation. Using the unique Vaisala PEROXCAP ® hydrogen peroxide sensor technology, the HPP272 probe can also measure two other parameters: dewpoint and vapor pressure, which can also be useful parameters in bio-decontamination. The probe guarantees reliable and precise hydrogen peroxide measurements throughout thebio-decontamination cycle, even in high humidity.The reliable and reproducible measurements of Vaisala’s vaporized hydrogen peroxide probes allow STERIS to achieve a high degree of confidence in their bio-decontamination procedures, success during annual audits, and a high level of product quality.Please contact us at/contactusScan the code formore informationRef. B212075EN-A ©Vaisala 2020This material is subject to copyright protection, with all copyrights retained by Vaisala and its individual partners. All rights reserved. Any logos and/or product names are trademarks of Vaisala or its individual partners. The reproduction, transfer, distribution or storage of information contained in this brochure in any form without the prior written consent of Vaisala is strictly prohibited. All specifications — technical included — are subject to change without notice.。
杏鲍菇废弃菌渣中D-氨基葡萄糖盐酸盐的制备工艺及生物学活性分析
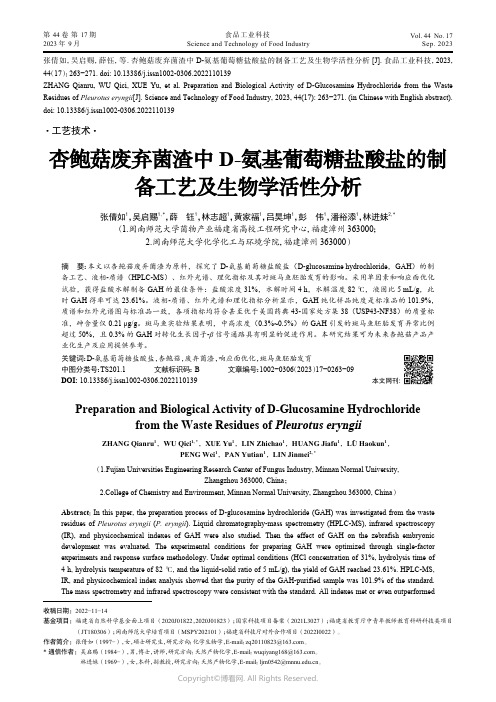
张倩如,吴启赐,薛钰,等. 杏鲍菇废弃菌渣中D-氨基葡萄糖盐酸盐的制备工艺及生物学活性分析[J]. 食品工业科技,2023,44(17):263−271. doi: 10.13386/j.issn1002-0306.2022110139ZHANG Qianru, WU Qici, XUE Yu, et al. Preparation and Biological Activity of D-Glucosamine Hydrochloride from the Waste Residues of Pleurotus eryngii [J]. Science and Technology of Food Industry, 2023, 44(17): 263−271. (in Chinese with English abstract).doi: 10.13386/j.issn1002-0306.2022110139· 工艺技术 ·杏鲍菇废弃菌渣中D-氨基葡萄糖盐酸盐的制备工艺及生物学活性分析张倩如1,吴启赐1, *,薛 钰1,林志超1,黄家福1,吕昊坤1,彭 伟1,潘裕添1,林进妹2,*(1.闽南师范大学菌物产业福建省高校工程研究中心,福建漳州 363000;2.闽南师范大学化学化工与环境学院,福建漳州 363000)摘 要:本文以杏鲍菇废弃菌渣为原料,探究了D-氨基葡萄糖盐酸盐(D-glucosamine hydrochloride ,GAH )的制备工艺、液相-质谱(HPLC-MS )、红外光谱、理化指标及其对斑马鱼胚胎发育的影响。
采用单因素和响应面优化试验,获得盐酸水解制备GAH 的最佳条件:盐酸浓度31%,水解时间4 h ,水解温度82 ℃,液固比5 mL/g ,此时GAH 得率可达23.61%。
液相-质谱、红外光谱和理化指标分析显示,GAH 纯化样品纯度是标准品的101.9%,质谱和红外光谱图与标准品一致,各项指标均符合甚至优于美国药典43-国家处方集38(USP43-NF38)的质量标准,砷含量仅0.21 μg/g 。
醋酸在制氢中缓冲作用
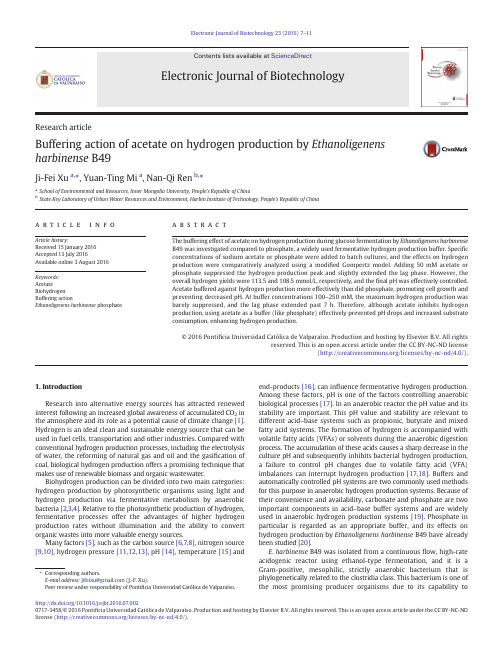
ResearcharticleBuffering action of acetate on hydrogen production by Ethanoligenens harbinense B49Ji-Fei Xu a ,⁎,Yuan-Ting Mi a ,Nan-Qi Ren b ,⁎a School of Environmental and Resources,Inner Mongolia University,People's Republic of ChinabState Key Laboratory of Urban Water Resources and Environment,Harbin Institute of Technology,People's Republic of Chinaa b s t r a c ta r t i c l e i n f o Article history:Received 15January 2016Accepted 13July 2016Available online 3August 2016The buffering effect of acetate on hydrogen production during glucose fermentation by Ethanoligenens harbinense B49was investigated compared to phosphate,a widely used fermentative hydrogen production buffer.Speci fic concentrations of sodium acetate or phosphate were added to batch cultures,and the effects on hydrogen production were comparatively analyzed using a modi fied Gompertz model.Adding 50mM acetate or phosphate suppressed the hydrogen production peak and slightly extended the lag phase.However,the overall hydrogen yields were 113.5and 108.5mmol/L,respectively,and the final pH was effectively controlled.Acetate buffered against hydrogen production more effectively than did phosphate,promoting cell growth and preventing decreased pH.At buffer concentrations 100–250mM,the maximum hydrogen production was barely suppressed,and the lag phase extended past 7h.Therefore,although acetate inhibits hydrogen production,using acetate as a buffer (like phosphate)effectively prevented pH drops and increased substrate consumption,enhancing hydrogen production.©2016Ponti ficia Universidad Católica de Valparaíso.Production and hosting by Elsevier B.V.All rightsreserved.This is an open access article under the CC BY-NC-ND license(/licenses/by-nc-nd/4.0/).Keywords:AcetateBiohydrogen Buffering actionEthanoligenens harbinense phosphate1.IntroductionResearch into alternative energy sources has attracted renewed interest following an increased global awareness of accumulated CO 2in the atmosphere and its role as a potential cause of climate change [1].Hydrogen is an ideal clean and sustainable energy source that can be used in fuel cells,transportation and other pared with conventional hydrogen production processes,including the electrolysis of water,the reforming of natural gas and oil and the gasi fication of coal,biological hydrogen production offers a promising technique that makes use of renewable biomass and organic wastewater.Biohydrogen production can be divided into two main categories:hydrogen production by photosynthetic organisms using light and hydrogen production via fermentative metabolism by anaerobic bacteria [2,3,4].Relative to the photosynthetic production of hydrogen,fermentative processes offer the advantages of higher hydrogen production rates without illumination and the ability to convert organic wastes into more valuable energy sources.Many factors [5],such as the carbon source [6,7,8],nitrogen source [9,10],hydrogen pressure [11,12,13],pH [14],temperature [15]andend-products [16],can in fluence fermentative hydrogen production.Among these factors,pH is one of the factors controlling anaerobic biological processes [17].In an anaerobic reactor the pH value and its stability are important.This pH value and stability are relevant to different acid –base systems such as propionic,butyrate and mixed fatty acid systems.The formation of hydrogen is accompanied with volatile fatty acids (VFAs)or solvents during the anaerobic digestion process.The accumulation of these acids causes a sharp decrease in the culture pH and subsequently inhibits bacterial hydrogen production,a failure to control pH changes due to volatile fatty acid (VFA)imbalances can interrupt hydrogen production [17,18].Buffers and automatically controlled pH systems are two commonly used methods for this purpose in anaerobic hydrogen production systems.Because of their convenience and availability,carbonate and phosphate are two important components in acid –base buffer systems and are widely used in anaerobic hydrogen production systems [19].Phosphate in particular is regarded as an appropriate buffer,and its effects on hydrogen production by Ethanoligenens harbinense B49have already been studied [20].E.harbinense B49was isolated from a continuous flow,high-rate acidogenic reactor using ethanol-type fermentation,and it is a Gram-positive,mesophilic,strictly anaerobic bacterium that is phylogenetically related to the clostridia class.This bacterium is one of the most promising producer organisms due to its capability toElectronic Journal of Biotechnology 23(2016)7–11⁎Corresponding authors.E-mail address:Jifeixu@ (J.-F.Xu).Peer review under responsibility of Ponti ficia Universidad Católica deValparaíso./10.1016/j.ejbt.2016.07.0020717-3458/©2016Ponti ficia Universidad Católica de Valparaíso.Production and hosting by Elsevier B.V.All rights reserved.This is an open access article under the CC BY-NC-ND license (/licenses/by-nc-nd/4.0/).Contents lists available at ScienceDirectElectronic Journal of Biotechnologyefficiently and rapidly generate hydrogen[11],and its characteristics make it an interesting target for physiological and genetic studies aiming to improve its metabolic properties and increase its productivity with respect to hydrogen.This microorganism produces ethanol as a major fermentation product,in addition to CO2,acetate and H2 [21,22,23].The addition of ethanol had little inhibitory effect on fermentative hydrogen production,and the addition of acetate had a strong inhibitory effect on glucose consumption,bacterial growth and hydrogen production of E.harbinese B49[24].The hydrogen production is affected by the accumulation of self-produced byproducts,and acetate is therefore regarded as having an inhibitory rather than a buffering action during fermentative hydrogen production by E.harbinense B49.The inhibitory effect of acetate has been studied to understand the hydrogen-producing characteristics of these cultures,but its buffering effect has not been explored.In this paper,the effects of acetate on hydrogen production by E.harbinense B49were investigated to examine the buffering action of acetate on this process.2.Materials and methods2.1.Microorganism and mediaThe hydrogen-producing strain E.harbinense B49(AF481148in EMBL)was isolated from a continuousflow,high-rate acidogenic reactor using ethanol-type fermentation and then identified as a novel Ethanoligenens strain[24].The strain was stored in our lab at−80°C and cultured at36°C at an initial pH of6.5under strict anaerobic conditions.Cells from stock cultures were transferred into50-mL volumes of sterilised growth medium and incubated at35°C.When the cells entered a logarithmic growth phase,5mL of the pre-cultured broth was inoculated into a100-mL serum bottle containing50mL of basal medium,and the culture was grown anaerobically at35°C with shaking at130rpm.The hydrogen production medium consisted of (in g/L):glucose10.0,yeast extract3.0,NH4Cl0.5,MgCl20.18,K2HPO4 1.5,NaH2PO44.2and L-cysteine0.5.The basal medium also contained 1%trace element solution,1%vitamin solution and0.2%resazurin.The cells were harvested at the end of the exponential phase and used as inocula for the batch experiments.2.2.Batch testsThe buffering activities and inhibitory effects of phosphate,acetate and ethanol on the hydrogen-producing performance of strain B49 were investigated using serum bottles as batch reactors.All of the batch-fermentation studies were performed in250-mL serum bottles with a120-mL working volume.The hydrogen production medium also contained sodium acetate(NaAC·3H2O,at0,50,100,150,200or 250mM)or phosphate(Na2HPO4×2H2O-KH2PO4,at0,50,100,150, 200or250mM).Three bottles were tested in parallel for each condition.All media were sterilised by autoclaving at121°C and 15psig for30min.Each bottle was then inoculated with5.0mL of strain B49cell suspension and incubated under non-controlled pH conditions in an air-bath shaker at36±1°C and135rpm.The biogas was sampled for biogas content analysis using a syringe,and a liquid sample was simultaneously taken from the bottles.All tests were run in triplicate.2.3.Analytical methods2.3.1.Cell growth analysisThe cell dry weight was determined by drying the cells for24h at80°C to a constant weight in a convection-type hot air oven (HPG-9145,China).2.3.2.Liquid samplesCells in the liquid cultures were pelleted by centrifugation at 8000rpm for5min at room temperature.The culture supernatant was filtered through a2.5-cm diameter,0.45-μm polytetrafluoroethylene filter,transferred to sterile1-mL Eppendorf tubes and frozen until analysis.Volatile fatty acids and ethanol were detected using a gas chromatography(GC)system(HP6890,Agilent Technologies,USA) and aflame-ionisation detector(FID).The temperatures of the glass columns and injections were145°C and175°C,respectively.The carrier gas was N2,and the packing material was FON(which contains polyethylene glycol and2-nitroterephthalic acid),obtained from Shimadzu,Inc.The glucose concentration in the culture was determined according to the protocol in a kit(GOD-PAP,Shanghai Rongsheng Biological Technology Corporation,China),and the pH was measured using a pHS-25acidity voltmeter according to standard methods.2.3.3.Biogas compositionBiogas production was measured using the water displacement method.The biogas composition from the bioreactor was measured using GC(HP4890,Agilent)on an instrument equipped with a thermal conductivity detector(TCD).A stainless steel column packed with molecular sieve5A was used to detect H2.Nitrogen was used as the carrier gas at a rate of25mL/min.3.Modeling the kinetic parametersThe cumulative hydrogen production data werefitted using a modified Gompertz equation[25,26]as a suitable model for describing the progress of cumulative hydrogen production in the batch experiment.H¼PÁexp−expRmPλ−tðÞþ1½Equation1in which H is the cumulative hydrogen production(in mL/L);P is the hydrogen production potential(in mL/L);R m is the maximum hydrogen production rate(as mL/L/h);λis the time of the lag phase(h);e is 2.7182;and t is the incubation time(h).Table1Glucose degradation,cell growth and terminal pH at various acetate or phosphate concentrations.Buffer con.(mM)Degradation rate(%)Biomass(mg/L)Terminal pHPhosphate Acetate Phosphate Acetate Phosphate Acetate097±1.297±1.2619.32±61.9623±61.5 3.75±0.03 3.75±0.03 50100±1.4100±1.1646.07±58.3916±75.4 4.15±0.03 4.73±0.03 10095±0.6100±0.6616.87±46.51044±71.4 4.60±0.03 5.07±0.03 15087±1.1100±0.9505.68±56.41138±61.2 5.35±0.03 5.22±0.03 20063±1.6100±1.4455.19±50.31166±80.3 6.05±0.03 5.33±0.03 25050±0.598±0.8420.24±44.91213±90.1 6.20±0.03 5.41±0.03 8J.-F.Xu et al./Electronic Journal of Biotechnology23(2016)7–114.Results and discussion4.1.Glucose degradation and cell growthThe glucose degradation ef ficiencies,cell growth and terminal pH values at various phosphate and acetate concentrations are illustrated in Table 1.In the tests of acetate,the glucose was almost completely degraded at the end of fermentation,achieving 97–100%total glucose degradation.In contrast to acetate,the addition of phosphate had an obvious inhibitory effect on glucose degradation.From 0to 50mM phosphate,the glucose degradation reached approximately 97–100%.When more phosphate was added beyond 100mM,the glucose degradation rates declined,achieving only 50%glucose degradation at 250mM.This result indicates that the addition of excess phosphate had a signi ficant negative in fluence on glucose degradation.In the phosphate tests,cell growth was improved with 50mM phosphate.However,as the phosphate concentration increased,cell growth was gradually inhibited.In contrast,the total cell weight increased as more acetate was added.This result was inconsistent with that of another research report,a discrepancy that may be due to differences in the composition of the hydrogen production medium [24].The initial pH in all tests was approximately 6.5.As shown in Table 1,at the end of hydrogen-producing fermentation,the terminal pH values of media supplemented with 50mM or 100mM phosphate were much higher than those of acetate-supplemented media.However,when the concentrations of phosphate and acetate exceeded 150mM,the terminal pH values of the acetate-supplemented media were much higher than those supplemented with phosphate,due to the buffer system of sodium acetate and acetate.These results indicated that acetate was able to promote the cell growth of E.harbinense B49and raise the terminal pH as a result of its enhanced buffering of the fermentative system.In contrast,although phosphatewas able to raise the terminal pH by buffering Na 2HPO 4·2H 2O-KH 2PO 4,it also restrained cell growth.4.2.Time course of hydrogen production pro files4.2.1.Under different phosphate concentration conditionsHydrogen production by E.harbinense B49was signi ficantly affected by the phosphate concentration of the medium.As shown in Fig.1and Table 2,a slight increase in the cumulative hydrogen yield could be achieved by increasing the phosphate buffer concentration from 0mM to 50mM.A maximum P max of 108.54mmol/L and R max of 18.39mmol/L/h were observed at phosphate buffer concentrations of 50mM and 100mM,respectively.Subsequently,P max and R max decreased gradually as the phosphate buffer concentration increased,most likely due to the negative effect of increased cytoplasmic osmotic pressure [27].The lag phase times of hydrogen production became longer as the phosphate concentration increased.The final pH also increased with increasing phosphate buffer concentrations,whereas lower phosphate buffer concentrations were associated with lower pH values.Similar to glucose consumption and cell growth,hydrogen production also peaked at 50mM phosphate,as shown in Table 1and Table 2.Different results were obtained in previous studies of Citrobacter sp.Y19[28]and Rhodopseudomonas palustris P4[29].No inhibitory effect of phosphate on cell growth was observed at concentrations between 0and 300mM.The maximum hydrogen yield was obtained at concentrations of 50and 140mM phosphate by R.palustris P4and Citrobacter sp.Y19,respectively.The present results indicate that the optimal phosphate concentration is 50mM for E.harbinense B49.At this concentration,the maximal yield of hydrogen was produced;the most glucose was exhausted;and the lag phase was relatively shorter.Similar results were reported for Clostridium beijerinckii Fanp3[30].4.2.2.Under different acetate concentration conditionsThe effects of acetate concentration on hydrogen production are shown in Fig.2and Table 2.The glucose in the media was completely exhausted after 48h of incubation irrespective of the acetate concentration.However,the addition of acetate had a considerable impact on the cumulative hydrogen pared with hydrogen production medium that did not include acetate,an increase in the cumulative hydrogen yield could be achieved by increasing the acetate buffer concentration to 50mM,and the addition of additional acetate extended the lag phase of hydrogen production.The maximum P max of 113.53mmol/L and R max of 12.56mmol/L/h occurred at an acetate buffer concentration of 50mM and in media with no acetate added,respectively.When the concentration of acetate was greater than 50mM,slight inhibition of hydrogen production occurred,and the P max and R max values decreased gradually with increasing acetate concentration.However,cell growth was inversely related to the hydrogen production rate and increased with increasing acetate,as shown in Table 1.20406080100120C u m u l a t i v e H 2 p r o d u c t i o n (m M )Time (h)Fig.1.Time course of hydrogen production pro files during fermentation of glucose under different phosphate concentration conditions.The lines represent data calculated using Gompertz equation.Table 2Fermentation characteristics for hydrogen production at various phosphate or acetate concentrations.Buffer con.(mM)P max (mmol/L)R max (mmol/L/h)λ(h)R 2PhosphateAcetate Phosphate Acetate Phosphate Acetate Phosphate Acetate 0108.14108.1412.8412.56 4.2 4.20.99860.998650108.54113.5313.9610.53 4.4 6.80.99790.9905100102.69106.5218.39 6.197.08.80.99840.9932150105.86103.849.69 6.307.911.40.99550.998820084.34104.027.74 6.1610.511.60.99590.997125018.01100.222.967.4514.213.40.99440.99419J.-F.Xu et al./Electronic Journal of Biotechnology 23(2016)7–114.3.The amount of volatile organic compound and hydrogenThe amount of acetate,ethanol and hydrogen at various phosphate and acetate concentrations are shown in Fig.3.The amount of ethanol were increased slightly with increasing phosphate or acetate concentration,the concentration of acetate and the volume of hydrogen were varied only slightly while the concentration of acetate was increased,but decreased dramatically while the concentration of phosphate was increased.In contrast to acetate,the addition of phosphate had an obvious inhibitory effect on acetate and hydrogen production.At 250mM acetate,the volume of hydrogen exceeded 100mM which achieved the maximum volume of hydrogen at 50mM acetate.However,at 250mM phosphate,the volume of hydrogen was less than 20mM which is one fifth of the maximum volume of hydrogen at phosphate.This result indicates that the addition of excess phosphate had a signi ficant negative in fluence on hydrogen production.Hydrogen production from glucose by hydrogen-producing microorganisms also yields volatile organic acids,such as acetic acid and butyric acid,which lower the pH of the media and slow hydrogenproduction [31].To minimise the effects of these organic acids on the pH,phosphate buffers composed of Na 2HPO 4and NaH 2PO 4or KH 2PO 4were used to control the pH.Acetic acid is mainly a product of fermentation;therefore,acetate has been regarded as an inhibitor of hydrogen production and has not been used to control pH.However,the present results indicate that acetate was able to control the pH during fermentative hydrogen production from glucose by E.harbinense B49.We also evaluated the ability of phosphate and acetate to control pH during fermentation,and we found that although both phosphate and acetate were able to control the pH through their buffering activity,acetate was a stronger buffer than phosphate until the concentrations exceeded 150mM.The final pH increased with increasing concentrations of acetate and phosphate,but their patterns of buffer activity may be different.Sodium acetate and acetate,which were produced during fermentative hydrogen production,formed a buffer that grew increasingly strong.Acetate was “internal buffer system ”,while phosphate was “external buffer system ”.5.ConclusionsThe addition of acetate had both inhibitory and buffering effects on hydrogen production from glucose by E.harbinense B49.Acetate was able to control the pH changes caused by fermentative hydrogen production and increased the yield of hydrogen.At an acetate concentration of 50mM,maximal hydrogen production of 113.5mmol/L was achieved.The inhibitory effect of acetate on hydrogen production was mainly due to an extended lag phase,and acetate slightly decreased the cumulative hydrogen volume when added at concentrations between 100and 250mM.Therefore,using acetate as a buffering supplement can control the pH and alleviate the acidi fication of the growth medium.Con flict of interestWe have no con flict of interest to declare.AcknowledgmentsThis research was supported by the National Natural Science Foundation of China (Grant No.51108226).An earlier version of this paper was presented at 20th World Hydrogen Energy Conference 2014.References[1]Kotay SM,Das D.Biohydrogen as a renewable energy resource —Prospects andpotentials.Int J Hydrog Energy 2008;33:258–63./10.1016/j.ijhydene.2007.07.031.[2]Das D,Veziroglu T.Hydrogen production by biological processes:A survey ofliterature.Int J Hydrog Energy 2001;26:13–28./10.1016/S0360-3199(00)00058–6.[3]Hallenbeck PC,Ghosh D.Advances in fermentative biohydrogen production:Theway forward?Trends Biotechnol 2009;27:287–97./10.1016/j.tibtech.2009.02.004.[4]Ren N,Guo W,Liu B,Cao G,Ding J.Biological hydrogen production by darkfermentation:Challenges and prospects towards scaled-up production.Curr Opin Biotechnol 2011;22:365–70./10.1016/j.copbio.2011.04.022.[5]Wang JL,Wan W.Factors in fluencing fermentative hydrogen production:A review.Int J Hydrog Energy 2009;34:799–811./10.1016/j.ijhydene.2008.11.015.[6]Kádár Z,Vrije T,Van Noorden GE,Budde MAW,Szengyel Z,Réczey K,et al.Yieldsfrom glucose,xylose,and paper sludge hydrolysate during hydrogen production by the extreme thermophile Caldicellulosiruptor saccharolyticus .Appl Biochem Biotechnol 2004;114:497–508./10.1385/ABAB:114:1-3:497.[7]Niel EWJ,Pieternel AMC,Stams AJM.Substrate and product inhibition of hydrogenproduction by the extreme thermophile.Caldicellulo-siruptor saccharolyticus .Biotechnol Bioeng 2003;81:255–62./10.1002/bit.10463.[8]Panagiotopoulos IA,Bakker RR,Budde MAW,de Brije T,Claaseen PAM,Koukios EG.Fermentative hydrogen production from pretreated biomass:A comparative study.Bioresour Technol 2009;100:6331–8./10.1016/j.biortech.2009.07.011.[9]Lin CY,Lay CH.Carbon/nitrogen-ratio effect on fermentative hydrogen productionby mixed micro flora.Int J Hydrog Energy 2004;29:41–5./10.1016/S0360-3199(03)00083-1.306090120150)M m (n o i t c u d o r p f o s d l e i Y Concentration of buffer (mM)Fig.3.The change of acetate,ethanol and hydrogen yield under different phosphate or acetate concentration conditions (P and A represented phosphate and acetate buffer,respectively.The yield of acetate subtracted the concentration of acetate-supplemented in the media under different acetate concentration conditions).612182430364248C u m u l a t i v e H 2 p r o d u c t i o n (m M )Time (h)Fig.2.Time course of hydrogen production pro files during fermentation of glucose under different acetate concentration conditions.The lines represent data calculated using Gompertz equation.10J.-F.Xu et al./Electronic Journal of Biotechnology 23(2016)7–11[10]Xu L,Ren N,Wang X,Jia Y.Biohydrogen production by Ethanoligenens harbinenseB49:Nutrient optimization.Int J Hydrog Energy2008;33:6962–7./10.1016/j.ijhydene.2008.09.005.[11]Mizuno O,Dinsdale R,Hawkes FR,Hawkes DL,Noike T.Enhancement of hydrogenproduction from glucose by nitrogen gas sparging.Bioresour Technol2000;73: 59–65./10.1016/S0960-8524(99)00130–3.[12]Kraemer JT,Bagley DM.Supersaturation of dissolved H2and CO2duringfermentative hydrogen production with N2sparging.Biotechnol Lett2006;28: 1485–91./10.1007/s10529-006-9114-7.[13]Kraemer JT,Bagley DM.Improving the yield from fermentative hydrogenproduction.Biotechnol Lett2007;29:685–95./10.1007/s10529-006-9299-9.[14]Fang HHP,Liu H.Effect of pH on hydrogen production from glucose by a mixedculture.Bioresour Technol2002;82:87–93./10.1016/S0960-8524(01)00110–9.[15]Yokoyama H,Waki M,Moriya N,Yasuda T,Tanaka Y,Haga K.Effect of fermentationtemperature on hydrogen production from cow waste slurry by using anaerobic mi-croflora within the slurry.Appl Microbiol Biotechnol2007;74:474–83./10.1007/s00253-006-0647-4.[16]Zheng XJ,Yu HQ.Inhibitory effects of butyrate on biological hydrogen productionwith mixed anaerobic cultures.J Environ Manage2005;74:65–70./10.1016/j.jenvman.2004.08.015.[17]Lin CY,Lay CH.Effects of carbonate and phosphate concentrations on hydrogenproduction using anaerobic sewage sludge microflora.Int J Hydrog Energy2004;29:275–81./10.1016/j.ijhydene.2003.07.002.[18]Castro-Villalobos MC,García-Morales JL,Fernández FJ.By-products inhibition effectson bio-hydrogen production.Int J Hydrog Energy2012;37:7077–83./10.1016/j.ijhydene.2011.12.032.[19]Lin PJ,Chang JS,Yang LH,Lin CY,Wu SY,Lee KS.Enhancing the performance ofpilot-scale fermentative hydrogen production by proper combinations of HRT and substrate concentration.Int J Hydrog Energy2011;36:14289–94./10.1016/j.ijhydene.2011.04.147.[20]Wang XJ,Ren NQ,Xiang WS,Guo WQ.Influence of gaseous end-productsinhibition and nutrient limitations on the growth and hydrogen production by hydrogen-producing fermentative bacterial B49.Int J Hydrog Energy2007;32: 748–54./10.1016/j.ijhydene.2006.08.003.[21]Ren NQ,Xu L,Zhang Y,Xu H,Wang X,Chen G.Dependence on iron and hydrogenproducing pathway for novel strain Ethanoligenens sp.B49.Acta Sci Circumst 2006;26:1643–50./10.3321/j.issn:0253-2468.2006.10.011.[22]Hallenbeck PC,Abo-Hashesh M,Ghosh D.Strategies for improving biologicalhydrogen production.Bioresour Technol2012;110:1–9./10.1016/j.biortech.2012.01.103.[23]Castro JF,Razmilic V,Gerdtzen ZP.Genome based metabolicflux analysis ofEthanoligenens harbinense for enhanced hydrogen production.Int J Hydrog Energy 2013;8:1297–306./10.1016/j.ijhydene.2012.11.007.[24]Tang J.Inhibitory effects of acetate and ethanol on biohydrogen production ofEthanoligenens harbinese B49.Int J Hydrog Energy2012;37:741–7./10.1016/j.ijhydene.2011.04.067.[25]Wang AJ,Ren N,Shi Y,Lee DJ.Bioaugmented hydrogen production frommicrocrystalline cellulose using co-culture Clostridium acetobutylicum X9 and Ethanoligenens harbinense B49.Int J Hydrog Energy2008;33:912–7./10.1016/j.ijhydene.2007.10.017.[26]Antonopoulou G,Gavala HN,Skiadas IV,Lyberatos G.Modeling of fermentativehydrogen production from sweet sorghum extract based on modified ADM1.Int J Hydrog Energy2012;37:191–208./10.1016/j.ijhydene.2011.09.081.[27]Nath K,Das D.Modeling and optimization of fermentative hydrogen production.Bioresour Technol2011;102:8569–81./10.1016/j.biortech.2011.03.108.[28]Oh YK,Seol EH,Kim JR,Park S.Fermentative biohydrogen production by a newchemoheterotrophic bacterium Citrobacter sp.Y19.Int J Hydrog Energy2003;28: 1353–9./10.1016/S0360-3199(03)00024–7.[29]Oh YK,Seol EH,Lee EY,Park S.Fermentative hydrogen production by a newchemoheterotrophic bacterium Rhodopseudomonas palustris P4.Int J Hydrog Energy 2002;27:1373-19./10.1016/S0360-3199(02)00100–3.[30]Pan CM,Fan YT,Zhao P,Hou HW.Fermentative hydrogen production by the newlyisolated Clostridium beijerinckii Fanp3.Int J hydrog Energy2008;33:5383–91./10.1016/j.ijhydene.2008.05.037.[31]Van Lier JB,Grolle KC,Frijters CT,Stams AJ,Lettinga G.Effects of acetate,propionate,and butyrate on the thermophilic anaerobic degradation of propionate by methanogenic sludge and defined cultures.Appl Environ Microbiol1993;59: 1003–11.11J.-F.Xu et al./Electronic Journal of Biotechnology23(2016)7–11。
苯环喹溴铵药理毒理研究进展
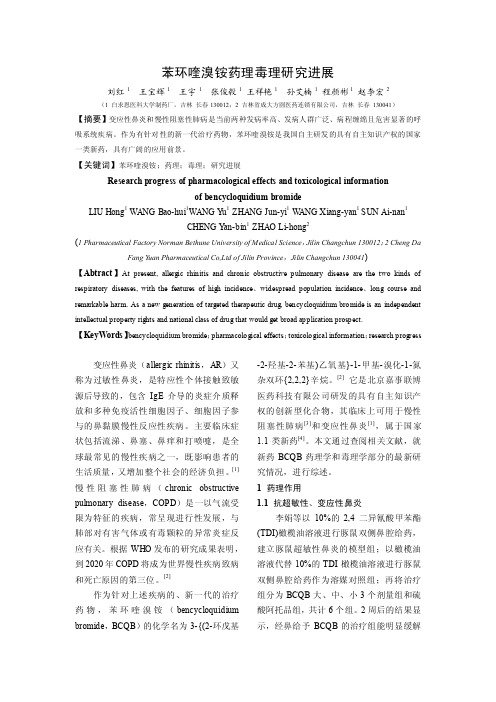
苯环喹溴铵药理毒理研究进展刘红1王宝辉1王宇1张俊毅1王祥艳1孙艾楠1程颜彬1赵李宏2(1白求恩医科大学制药厂,吉林长春130012;2吉林省成大方圆医药连锁有限公司,吉林长春130041)【摘要】变应性鼻炎和慢性阻塞性肺病是当前两种发病率高、发病人群广泛、病程缠绵且危害显著的呼吸系统疾病。
作为有针对性的新一代治疗药物,苯环喹溴铵是我国自主研发的具有自主知识产权的国家一类新药,具有广阔的应用前景。
【关键词】苯环喹溴铵;药理;毒理;研究进展Research progress of pharmacological effects and toxicological informationof bencycloquidium bromideLIU Hong1 WANG Bao-hui1WANG Y u1 ZHANG Jun-yi1 W ANG Xiang-yan1 SUN Ai-nan1CHENG Y an-bin1 ZHAO Li-hong2(1 Pharmaceutical Factory Norman Bethune University of Medical Science,Jilin Changchun 130012;2 Cheng DaFang Y uan Pharmaceutical Co,Ltd of Jilin Province,Jilin Changchun 130041)【Abtract】At present, allergic rhinitis and chronic obstructive pulmonary disease are the two kinds of respiratory diseases, with the features of high incidence、widespread population incidence、long course and remarkable harm. As a new generation of targeted therapeutic drug, bencycloquidium bromide is an independent intellectual property rights and national class of drug that would get broad application prospect. 【KeyWords】bencycloquidium bromide;pharmacological effects;toxicological information;research progress变应性鼻炎(allergic rhinitis,AR)又称为过敏性鼻炎,是特应性个体接触致敏源后导致的,包含IgE介导的炎症介质释放和多种免疫活性细胞因子、细胞因子参与的鼻黏膜慢性反应性疾病。
百普乐(培哚普利吲达帕胺片)

血液系统 - 极少发生(< 1/10, 000):
- 血小板减少症、白细胞减少症、粒性白血球缺乏症、再生障碍性贫血、溶血性贫血。 - 在特殊情况下(肾移植或进行血液透析的患者)服用血管紧张素转换酶抑制剂时可出现贫血(见注意事项)。
与吲达帕胺相关: 单独使用吲达帕胺治疗,具有可持续 24 小时的抗高血压作用。这一作用在其利尿作用尚很轻微的剂量下即可出现。
其抗高血压作用与其对动脉顺应性的改善和总动脉血管阻力及血管外周阻力的下降相平行。吲达帕胺可减轻左心室肥厚。
当使用噻嗪类利尿剂和与噻嗪类有关的利尿剂超过某一剂量后,抗高血压作用达到一个平台期,而不良反应将继续增加。因此如治疗效果不佳,不应增 加剂量。
[禁忌]
与培哚普利相关: 本品不用于下列情况: - 对培哚普利或其它任何血管紧张素转换酶抑制剂过敏 - 与使用血管紧张素转换酶抑制剂相关的血管神经性水肿(奎根水肿)的既往病史 - 遗传性或特发性血管神经性水肿 - 妊娠 - 哺乳
通常,本品不推荐用于: - 联合使用保钾性利尿剂, 钾盐,锂(见药物相互作用) - 双侧肾动脉狭窄或单肾。 - 高钾血症。
● 血管神经性水肿(奎根水肿) 已有报道服用血管紧张素转换酶抑制剂, 包括培哚普利治疗的患者,极少病例出现血管神经性水肿, 表现在面部、肢体、唇、舌、声门和 / 或喉部。如出 现这种情况,应立即停用培哚普利,并对患者实行监护直到水肿消退。
当水肿只出现在面部和唇部,一般不经治疗即可消退,但是可以使用抗组胺药以缓解症状。
[适应症]
原发性高血压。
[用法用量]
口服。 每日一次,每次服用一片百普乐, 最好在清晨餐前服用。血压不能控制时剂量可以加倍,每日二片百普乐或每日一片百普特。
重庆大学文献检索与利用1
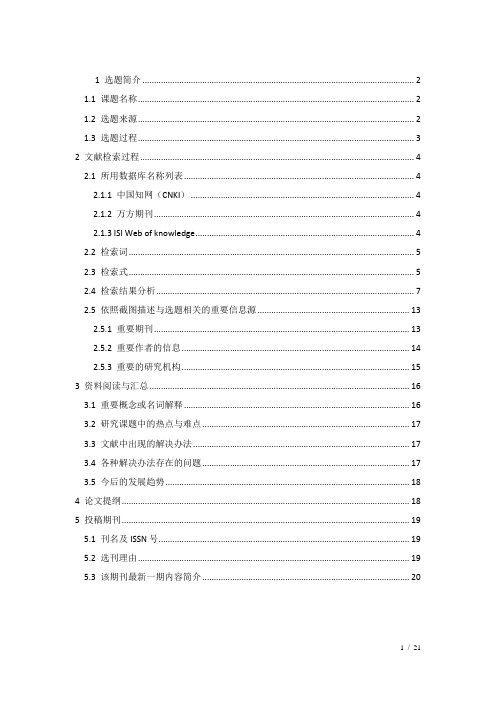
1 选题简介 (2)1.1 课题名称 (2)1.2 选题来源 (2)1.3 选题过程 (3)2 文献检索过程 (4)2.1 所用数据库名称列表 (4)2.1.1 中国知网(CNKI) (4)2.1.2 万方期刊 (4)2.1.3 ISI Web of knowledge (4)2.2 检索词 (5)2.3 检索式 (5)2.4 检索结果分析 (7)2.5 依照截图描述与选题相关的重要信息源 (13)2.5.1 重要期刊 (13)2.5.2 重要作者的信息 (14)2.5.3 重要的研究机构 (15)3 资料阅读与汇总 (16)3.1 重要概念或名词解释 (16)3.2 研究课题中的热点与难点 (17)3.3 文献中出现的解决办法 (17)3.4 各种解决办法存在的问题 (17)3.5 今后的发展趋势 (18)4 论文提纲 (18)5 投稿期刊 (19)5.1 刊名及ISSN号 (19)5.2 选刊理由 (19)5.3 该期刊最新一期内容简介 (20)1 选题简介1.1 课题名称厌氧发酵制氢的影响因素1.2 选题来源兴趣选题众所周知,能源是提高人民生活质量的主要物质基础,是制约国民经济发展的重要因素,同时也是维护国家安全的战略保障。
当今社会最广泛使用的能源主要是煤、石油、天然气和水力,特别是石油和天然气的消耗量增长迅速,已占全世界能源消费的60%左右。
然而化石能源价格持续上涨,能源短缺日益困扰国家和政府的发展规划,能源问题已成为影响国民经济发展和国家安全的重中之重;同时化石能源的使用对环境造成了严重的影响和破坏,温室效应、酸雨、臭氧层空洞等问题严重地威胁着地球和人类的可持续发展[1,2]。
因此从国家能源安全和环境保护角度考虑,改变能源生产和消费方式,促使人们开发环境友好和经济适用的可再生能源已经迫在眉睫。
2000年9月11日至15日,首届全球替代能源大会在德国慕尼黑举行,与会代表们强烈呼吁各国政府和公民从现在开始真正认识到替代能源的重要性和紧迫性。
百普乐(培哚普利吲达帕胺片)

特殊警告 与培哚普利相关:
● 在免疫功能低下患者发生中性白细胞减少症 / 粒细胞缺乏症的危险 中性粒细胞减少症的危险与剂量及患者类型相关,并取决于患者的临床情况。没有并发症的患者极少会出现这种情况,但是与胶原血管性疾病相关的肾 功能不全的患者可能发生,如系统性红斑狼疮或硬皮病患者以及使用免疫抑制剂治疗的患者。 停止使用血管紧张素转化酶抑制剂治疗,危险性可消失。 严格遵守预先规定的剂量用药可能是防止事件发生的最好办法。但是,如果这些患者需要使用血管紧张素转化酶抑制剂,应慎重评估风险 / 效益比值。
儿童 百普乐不能用于儿童,因为儿童单独应用或联合应用培哚普利的疗效和耐受性尚未确定。
[不良反应]
服用培哚普利可抑制肾素-血管紧张素-醛固酮轴而使吲达帕胺所致的失钾减少。服用百普乐的 2%患者出现低钾血症(钾离子水平< 3.4mmol/l)。
胃肠道 - 通常发生(> 1/100, < 1/10):便秘、口干、恶心、上腹痛、厌食、腹痛、味觉障碍。 - 极少发生(< 1/10, 000):胰腺炎。 - 在肝功能不全病例中,有引发肝性脑病的可能性(见禁忌和注意事项)。
一个月内血压即出现下降,无急速抗药反应;停药后无反弹作用。在临床试验中,同时给予培哚普利和吲达帕胺,与分别单独使用这二种药物相比,可 产生具有协同作用的抗高血压疗效。
与培哚普利相关: 培哚普利可以治疗各种程度的高血压:轻度到中度或重度。可以降低卧位和立位的收缩压和舒张压。 最大降压作用出现在服用单一剂量后 4-6 小时,降压作用可持续 24 小时以上。
培哚普利在低或正常肾素水平的患者中也可产生抗高血压作用。
培哚普利通过它的活性代谢产物—培哚普利拉产生作用。其它的代谢产物均无活性。
培哚普利可减轻心脏负荷: - 通过改变前列腺素代谢产生扩张静脉的作用:减轻前负荷, - 通过降低总外周血管阻力:减轻后负荷。
碧云天生物技术碘化丙啶产品说明书

碧云天生物技术/Beyotime Biotechnology订货热线:400-168-3301或800-8283301订货e-mail:******************技术咨询:*****************网址:碧云天网站微信公众号Propidium Iodide/碘化丙啶产品编号产品名称包装ST512 Propidium Iodide/碘化丙啶20mg产品简介:Propidium Iodide简称PI,中文名为碘化丙啶。
分子式为C27H34I2N4,分子量为668.40,纯度>95%。
进口分装,常用于细胞凋亡(apoptosis)或细胞坏死(necrosis)的检测,常用于流式细胞仪分析。
包装清单:产品编号产品名称包装ST512 Propidium Iodide/碘化丙啶20mg—说明书1份保存条件:4ºC避光保存。
注意事项:本产品对人体有刺激性,操作时请小心,并注意适当防护以避免直接接触人体或吸入体内。
本产品仅限于专业人员的科学研究用,不得用于临床诊断或治疗,不得用于食品或药品,不得存放于普通住宅内。
为了您的安全和健康,请穿实验服并戴一次性手套操作。
使用本产品的文献:1.Wu G, Liu ZS, Qian Q, Jiang CQ. Effects of Berberine on the Growth ofHepatocellular Carcinoma Cell lines. Medical Journal of Wuhan University. 2008 Jan;29(1):102-105.2.Liu Y, Sheng Z, Liu H, Wen D, He Q, Wang S, Shao W, Jiang RJ, An S,Sun Y, Bendena WG, Wang J, Gilbert LI, Wilson TG, Song Q, Li S.Juvenile hormone counteracts the bHLH-PAS transcription factors MET and GCE to prevent caspase-dependent programmed cell death in Drosophila. Development. 2009 Jun;136(12):2015-25.3.Li DL, Liu JJ, Liu BH, Hu H, Sun L, Miao Y, Xu HF, Yu XJ, Ma X, RenJ, Zang WJ. Acetylcholine inhibits hypoxia-induced tumor necrosis factor-α production via regulation of MAPKsphosphorylation in cardiomyocytes. J Cell Physiol. 2011 Apr;226(4):1052-9.4.Cao X, Deng W, Wei Y, Su W, Yang Y, Wei Y, Yu J, Xu X. Encapsulationof plasmid DNA in calcium phosphate nanoparticles: stem cell uptake and genetransfer efficiency. Int J Nanomedicine. 2011;6:3335-49.5.Meng LY, Liu HR, Shen Y, Yu YQ, Tao X. Cochinchina momordica seedextract induces G2/M arrest and apoptosis in human breast cancerMDA-MB-231 cells by modulating the PI3K/Akt pathway. Asian Pac J Cancer Prev. 2011;12(12):3483-8.6.Zhao Q, Xue Y, Wang JF, Li H, Long TT, Li Z, Wang YM, Dong P, XueCH. In vitro and in vivo anti-tumour activities of echinoside A and ds-echinoside A from Pearsonothuriagraeffei. J Sci Food Agric. 2012 Mar 15;92(4):965-74.7.Tu Z, Ma Y, Tian J, Li H, Akers W, Achilefu S, Gu Y. Estrogen receptor βpotentiates the antiproliferative effect of raloxifene and affects the cellmigration and invasion in HCT-116 colon cancer cells. J Cancer Res Clin Oncol. 2012 Jul;138(7):1091-103.8.Zhou Z, Wan Y, Zhang Y, Wang Z, Jia R, Fan Y, Nie H, Ying S, Huang P,Wang F. Follicular development and expression of nuclear respiratory factor-1 and peroxisome proliferator-activated receptor γ coactivator-1 alpha in ovaries of fetal and neonatal doelings. J Anim Sci. 2012 Nov;90(11):3752-61.9.Jun Fang, Meihu Ma, Yongguo Jin, Ning Qiu, Chan Wang, Guodong Renand Xin Huang. Assessment of Salmonella enteritidis Viability in Egg White during Early Incubation Stages by Fluorescent Staining Method.Asian Journal of Animal and Veterinary Advances. 7: 556-67.10.Zhen-Jun S, Yuan-Yuan Z, Ying-Ying F, Shao-Ju J, Jiao Y, Xiao-Wei Z,Jian C, Yao X, Li-Ming Z.β,β-Dimethylacrylshikonin exerts antitumoractivity via Notch-1 signaling pathway in vitro and invivo. Biochem Pharmacol. 2012 Aug 15;84(4):507-12.11.Tu Z, Li H, Ma Y, Tang B, Tian J, Akers W, Achilefu S, Gu Y. Theenhanced ant iproliferative response to combined treatment of trichostatin A with raloxifene in MCF-7 breast cancer cells and its relevance to estro gen receptor β expression. Mol Cell Biochem. 2012 Jul;366(1-2):111-22.12.Feng C, Xu Z, Li Z, Zhang D, Liu Q, Lu L. Down-regulation of Wnt10aby RNA interference inhibits proliferation and promotes apoptosis in mouse embryonic palatal mesenchymal cells throu gh Wnt/β-catenin signaling pathway. J Physiol Biochem. 2013 Dec;69(4):855-63.13.Hou Y, Chu M, Du FF, Lei JY, Chen Y, Zhu RY, Gong XH, Ma X, Jin J.Recombinant disintegrin domain of ADAM15 inhibits the proliferation and migration of Bel-7402 cells. Biochem Biophys Res Commun. 2013 Jun 14;435(4):640-5.14.Li Q, Zhou X, Shi Y, Li J, Zheng L, Cui L, Zhang J, Wang L, Han Z, HanY, Fan D. In vivo tracking and comparison of the therapeutic effects of MSCs and HSCs for liver injury. PLoS One. 2013 Apr 30;8(4):e62363. 15.Zhou R, Huang W, Yao Y, Wang Y, Li Z, Shao B, Zhong J, Tang M,Liang S, Zhao X, Tong A, Yang J.CA II, a potential biomarker by proteomic analysis, exerts significant inhibitory effect on the growth of colorectal cancer cells. Int J Oncol. 2013 Aug;43(2):611-21.16.Wang Y, Jiang XL, Peng SW, Guo XY, Shang GG, Chen JC, Wu Q, ChenGQ. Induced apoptosis of osteoblasts proliferating on polyhydroxyal kanoates. Biomaterials. 2013 May;34(15):3737-46.17.Yang F, Huang W, Li Y, Liu S, Jin M, Wang Y, Jia L, Gao Z. Anti-tumoreffects in mice induced by survivin-targeted siRNA delivered through polysaccharide nanoparticles. Biomaterials. 2013 Jul;34(22):5689-99.18.Zhou S, Wu H, Zeng C, Xiong X, Tang S, Tang Z, Sun X. ApolipoproteinE protects astrocytes from hypoxia and glutamate-induced apoptosis.FEBS Lett. 2013 Jan 16;587(2):254-8.19.Li R, Luo X, Li L, Peng Q, Yang Y, Zhao L, Ma M, Hou Z. TheProtective Effects of Melatonin Against Oxidative Stress and Inflammation Induced by AcuteCadmium Exposure in Mice Testis. Biol Trace Elem Res. 2016 Mar;170(1):152-64.Version 2016.12.08。
不同酶消化法提取猪原代肝细胞的效果比较
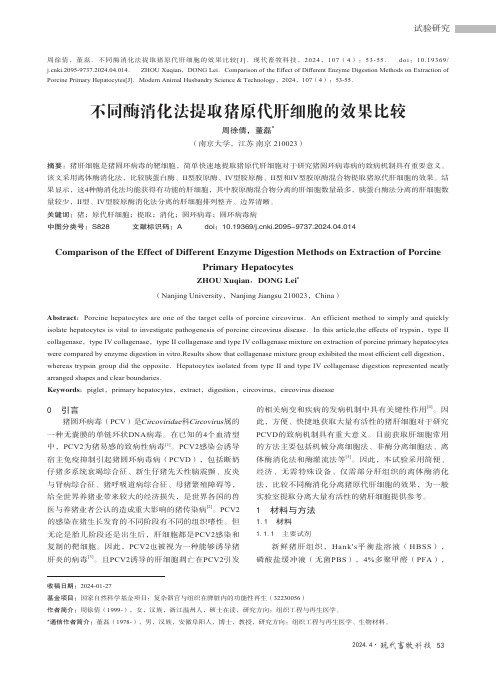
532024.4·试验研究0 引言猪圆环病毒(PCV )是Circoviridae 科Circovirus 属的一种无囊膜的单链环状DNA 病毒。
在已知的4个血清型中,PCV2为猪易感的致病性病毒[1]。
PCV2感染会诱导宿主免疫抑制引起猪圆环病毒病(PCVD ),包括断奶仔猪多系统衰竭综合征、新生仔猪先天性脑震颤、皮炎与肾病综合征、猪呼吸道病综合征、母猪繁殖障碍等,给全世界养猪业带来较大的经济损失,是世界各国的兽医与养猪业者公认的造成重大影响的猪传染病[2]。
PCV2的感染在猪生长发育的不同阶段有不同的组织嗜性。
但无论是胎儿阶段还是出生后,肝细胞都是PCV2感染和复制的靶细胞。
因此,PCV2也被视为一种能够诱导猪肝炎的病毒[3]。
且PCV2诱导的肝细胞凋亡在PCV2引发的相关病变和疾病的发病机制中具有关键性作用[4]。
因此,方便、快捷地获取大量有活性的猪肝细胞对于研究PCVD 的致病机制具有重大意义。
目前获取肝细胞常用的方法主要包括机械分离细胞法、非酶分离细胞法、离体酶消化法和酶灌流法等[5]。
因此,本试验采用简便、经济、无需特殊设备、仅需部分肝组织的离体酶消化法,比较不同酶消化分离猪原代肝细胞的效果,为一般实验室提取分离大量有活性的猪肝细胞提供参考。
1 材料与方法1.1 材料1.1.1 主要试剂新鲜猪肝组织,Hank's 平衡盐溶液(HBSS ),磷酸盐缓冲液(无菌PBS ),4%多聚甲醛(PFA ),收稿日期:2024-01-27基金项目:国家自然科学基金项目:复杂器官与组织在脾脏内的功能性再生(32230056)作者简介:周徐倩(1999-),女,汉族,浙江温州人,硕士在读,研究方向:组织工程与再生医学。
*通信作者简介:董磊(1978-),男,汉族,安徽阜阳人,博士,教授,研究方向:组织工程与再生医学、生物材料。
周徐倩,董磊.不同酶消化法提取猪原代肝细胞的效果比较[J].现代畜牧科技,2024,107(4):53-55. doi :10.19369/ki.2095-9737.2024.04.014. ZHOU Xuqian ,DONG Lei .Comparison of the Effect of Different Enzyme Digestion Methods on Extraction of Porcine Primary Hepatocytes[J].Modern Animal Husbandry Science & Technology ,2024,107(4):53-55.不同酶消化法提取猪原代肝细胞的效果比较周徐倩,董磊*(南京大学,江苏 南京 210023)摘要:猪肝细胞是猪圆环病毒的靶细胞,简单快速地提取猪原代肝细胞对于研究猪圆环病毒病的致病机制具有重要意义。
世界卫生组织儿童标准处方集
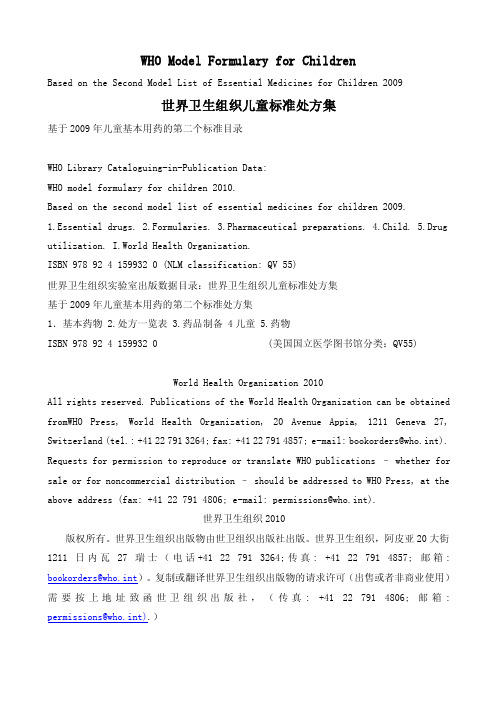
WHO Model Formulary for ChildrenBased on the Second Model List of Essential Medicines for Children 2009世界卫生组织儿童标准处方集基于2009年儿童基本用药的第二个标准目录WHO Library Cataloguing-in-Publication Data:WHO model formulary for children 2010.Based on the second model list of essential medicines for children 2009.1.Essential drugs.2.Formularies.3.Pharmaceutical preparations.4.Child.5.Drug utilization. I.World Health Organization.ISBN 978 92 4 159932 0 (NLM classification: QV 55)世界卫生组织实验室出版数据目录:世界卫生组织儿童标准处方集基于2009年儿童基本用药的第二个标准处方集1.基本药物 2.处方一览表 3.药品制备 4儿童 5.药物ISBN 978 92 4 159932 0 (美国国立医学图书馆分类:QV55)World Health Organization 2010All rights reserved. Publications of the World Health Organization can be obtained fromWHO Press, World Health Organization, 20 Avenue Appia, 1211 Geneva 27, Switzerland (tel.: +41 22 791 3264; fax: +41 22 791 4857; e-mail: ******************). Requests for permission to reproduce or translate WHO publications – whether for sale or for noncommercial distribution – should be addressed to WHO Press, at the aboveaddress(fax:+41227914806;e-mail:*******************).世界卫生组织2010版权所有。
吩噻嗪类衍生物
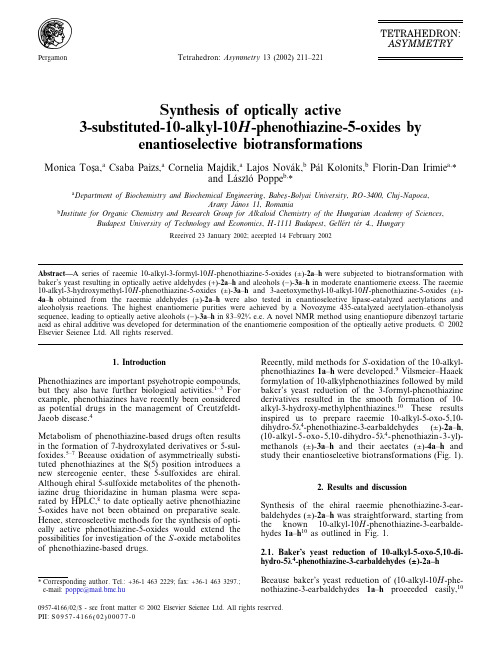
1. Introduction Phenothiazines are important psychotropic compounds, but they also have further biological activities.1–3 For example, phenothiazines have recently been considered as potential drugs in the management of CreutzfeldtJacob disease.4 Metabolism of phenothiazine-based drugs often results in the formation of 7-hydroxylated derivatives or 5-sulfoxides.5–7 Because oxidation of asymmetrically substituted phenothiazines at the S(5) position introduces a new stereogenic center, these 5-sulfoxides are chiral. Although chiral 5-sulfoxide metabolites of the phenothiazine drug thioridazine in human plasma were separated by HPLC,8 to date optically active phenothiazine 5-oxides have not been obtained on preparative scale. Hence, stereoselective methods for the synthesis of optically active phenothiazine-5-oxides would extend the possibilities for investigation of the S -oxide metabolites of phenothiazine-based drugs.
生物制氢原理英语作文
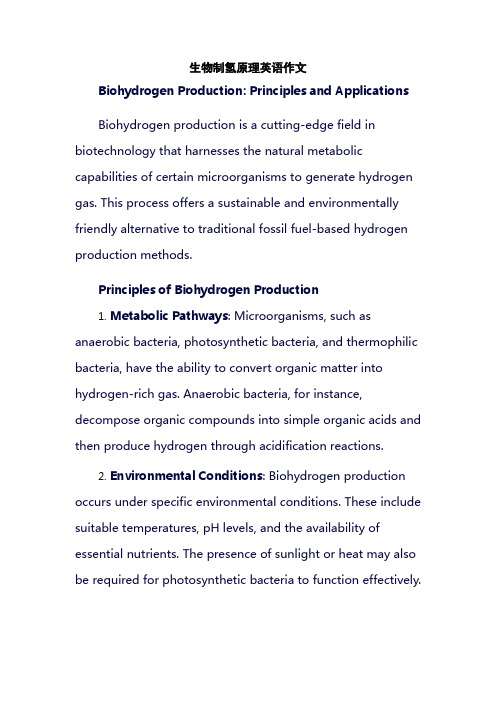
生物制氢原理英语作文Biohydrogen Production: Principles and ApplicationsBiohydrogen production is a cutting-edge field in biotechnology that harnesses the natural metabolic capabilities of certain microorganisms to generate hydrogen gas. This process offers a sustainable and environmentally friendly alternative to traditional fossil fuel-based hydrogen production methods.Principles of Biohydrogen Production1.Metabolic Pathways: Microorganisms, such as anaerobic bacteria, photosynthetic bacteria, and thermophilic bacteria, have the ability to convert organic matter into hydrogen-rich gas. Anaerobic bacteria, for instance, decompose organic compounds into simple organic acids and then produce hydrogen through acidification reactions.2.Environmental Conditions: Biohydrogen production occurs under specific environmental conditions. These include suitable temperatures, pH levels, and the availability of essential nutrients. The presence of sunlight or heat may also be required for photosynthetic bacteria to function effectively.3.Biomass Utilization: Organic waste or biomass, such as agricultural residues and industrial effluents, can be utilized as feedstocks for biohydrogen production. This not only reduces the cost of production but also contributes to waste management and resource recovery.Advantages of Biohydrogen Production1.Environmental Friendliness: Unlike fossil fuels, biohydrogen does not release harmful greenhouse gases when burned. It produces only water as a byproduct, making ita truly "zero-emission" energy source.2.Renewability: As biohydrogen is derived from renewable organic matter, it can be produced indefinitely provided there is a constant supply of biomass. This ensures its long-term sustainability.3.Energy Efficiency: Hydrogen has a high energy density, with a thermal value of 143 MJ/kg. This is approximately three times the heat value of oil, making it an energy-efficient fuel.ConclusionIn conclusion, biohydrogen production represents a promising solution to address the global energy crisis and environmental concerns. Its principles, based on microbialmetabolic pathways, offer a sustainable and environmentally friendly alternative to traditional hydrogen production methods. With the availability of organic waste and biomass as feedstocks, biohydrogen production has the potential to contribute significantly to waste management, resource recovery, and energy security.。
鞘氨醇单胞菌发酵生产韦兰胶的研究
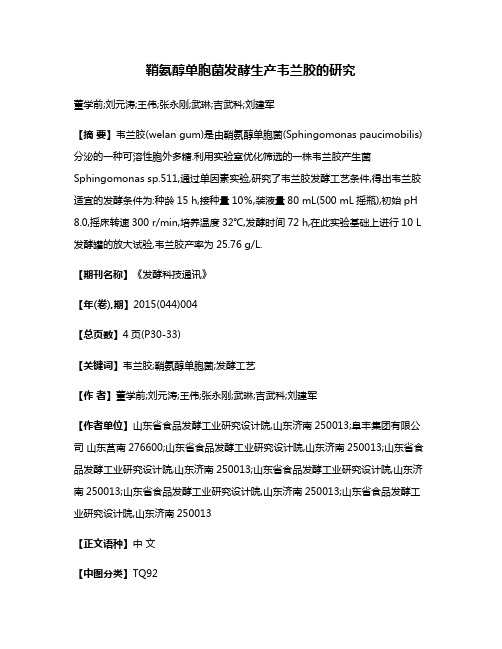
鞘氨醇单胞菌发酵生产韦兰胶的研究董学前;刘元涛;王伟;张永刚;武琳;吉武科;刘建军【摘要】韦兰胶(welan gum)是由鞘氨醇单胞菌(Sphingomonas paucimobilis)分泌的一种可溶性胞外多糖.利用实验室优化筛选的一株韦兰胶产生菌Sphingomonas sp.511,通过单因素实验,研究了韦兰胶发酵工艺条件,得出韦兰胶适宜的发酵条件为:种龄15 h,接种量10%,装液量80 mL(500 mL摇瓶),初始pH 8.0,摇床转速300 r/min,培养温度32℃,发酵时间72 h,在此实验基础上进行10 L 发酵罐的放大试验,韦兰胶产率为25.76 g/L.【期刊名称】《发酵科技通讯》【年(卷),期】2015(044)004【总页数】4页(P30-33)【关键词】韦兰胶;鞘氨醇单胞菌;发酵工艺【作者】董学前;刘元涛;王伟;张永刚;武琳;吉武科;刘建军【作者单位】山东省食品发酵工业研究设计院,山东济南250013;阜丰集团有限公司山东莒南276600;山东省食品发酵工业研究设计院,山东济南250013;山东省食品发酵工业研究设计院,山东济南250013;山东省食品发酵工业研究设计院,山东济南250013;山东省食品发酵工业研究设计院,山东济南250013;山东省食品发酵工业研究设计院,山东济南250013【正文语种】中文【中图分类】TQ92韦兰胶(welan gum)是由鞘氨醇单胞菌(Sphingomonas sp.)分泌的一种可溶性胞外多聚糖,其主链结构由D-葡萄糖、D-葡萄糖醛酸、D-葡萄糖和L-鼠李糖重复单元构成,侧链由单一的L-吡喃鼠李糖基或L-吡喃甘露糖基构成[1-2],此外约半数的主链重复单元含有乙酰基及甘油基团[3-4],平均分子量在400~2 000 kDa之间[5]。
韦兰胶溶液是一种典型的假塑性流体,与黄原胶相比,同等浓度下水溶液黏度高,特别是在低浓度下能保持较好的黏度;耐酸碱能力强,在pH 2~12范围内黏度几乎保持不变;温度对韦兰胶溶液的黏度影响较小[6-7],0.4%的黄原胶溶液在135℃时黏度已趋于零,但同等条件下韦兰胶溶液在163℃时黏度才接近于零。
金担子素A
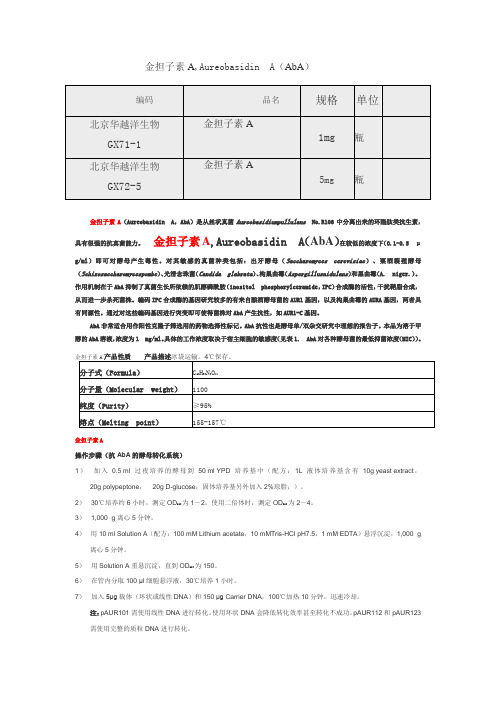
金担子素A ,Aureobasidin A (AbA )金担子素A (Aureobasidin A ,AbA )是从丝状真菌Aureobasidiumpullulans No.R106中分离出来的环酯肽类抗生素,具有很强的抗真菌能力。
金担子素A ,Aureobasidin A (AbA )在较低的浓度下(0.1-0.5 μg/ml )即可对酵母产生毒性。
对其敏感的真菌种类包括:出牙酵母(Saccharomyces cerevisiae )、粟酒裂殖酵母(Schizosaccharomycespombe )、光滑念珠菌(Candida glabrata )、构巢曲霉(Aspergillusnidulans )和黑曲霉(A. niger.)。
作用机制在于AbA 抑制了真菌生长所依赖的肌醇磷酰胺(inositol phosphorylceramide ,IPC )合成酶的活性,干扰鞘脂合成,从而进一步杀死菌株。
编码IPC 合成酶的基因研究较多的有来自酿酒酵母菌的AUR1基因,以及构巢曲霉的AURA 基因,两者具有同源性。
通过对这些编码基因进行突变即可使得菌株对AbA 产生抗性,如AUR1-C 基因。
AbA 非常适合用作阳性克隆子筛选用的药物选择性标记。
AbA 抗性也是酵母单/双杂交研究中理想的报告子。
本品为溶于甲醇的AbA 溶液,浓度为1 mg/ml 。
具体的工作浓度取决于宿主细胞的敏感度(见表1. AbA 对各种酵母菌的最低抑菌浓度(MIC ))。
金担子素A操作步骤(抗AbA 的酵母转化系统)1) 加入0.5 ml 过夜培养的酵母到50 ml YPD 培养基中(配方:1L 液体培养基含有10g yeast extract ,20g polypeptone , 20g D-glucose ;固体培养基另外加入2%琼脂;)。
2) 30℃培养约6小时,测定OD 660为1~2。
使用二倍体时,测定OD 660为2~4。
杀菌剂肟菌酯的合成

杀菌剂肟菌酯的合成肟菌酯(Trifloxystrobin)是甲氧基丙烯酸酯类杀菌剂,随着此类天然β-甲氧基丙烯酸酯衍生物的发现,人们研发出了新一类广谱、高效、低毒的杀菌剂。
肟菌酯的开发为世界农药市场注入了新鲜的活力,该杀菌剂性能卓越、防效持久而且杀菌方式独特,所以其市场潜力巨大。
另外,Bostanian 等通过大量动物实验和研究,发现肟菌酯毒性极小,如若被吸收进体内,大部分都会被排出体外,只会有极小的残留。
对肟菌酯进行药理实验,发现它对胎仔无催畸形性,无突变异性,所以该杀菌剂安全性高,而且肟菌酯溶解快、易水解,也能在植物体内、土壤以及水中迅速降解,对动植物毒性极低,对环境友好,堪称绿色杀菌剂。
1 肟菌酯传统合成工艺的概述1993年Brand等申请了一篇专利,专利中以邻甲基苯乙酸甲酯为原料,使用液溴溴化得到邻溴甲基苯乙酸甲酯,再让它与(E)-间三氟甲基苯乙酮肟反应得到(E)-2-(α-(((α-甲基-3-三氟甲基苯基)亚胺)氧)-O-甲苯基)-乙酸甲酯,接着再将其氧化,之后与甲氧基胺盐酸盐反应,最后得到目标产物肟菌酯。
对于第一步反应,有文献选择溴化,也有文献选择氯化,因为氯代在工业上比较便宜,但是氯的离去性显然没有溴好,不易发生取代反应。
Cliff等就使用NBS在四氯化碳中进行溴化反应,产率也比较理想。
1995年Ziegler等以邻溴甲基苯硼酸为起始原料,将它跟(E)-间三氟甲基苯乙酮肟进行反应,得到相应的取代产物后再和2-氯-2-甲氧基亚胺-乙酸甲酯反应,即可得到肟菌酯。
该方法最后一步使用到了昂贵的催化剂Pd(PPh3)4,成本较高,不适合工业化。
1996年Pfiffner等使用邻溴甲基苯乙酸甲酯与苯甲酰羟胺作为起始原料,在碱性条件下脱去一分子HBr,产物再进一步在盐酸作用下得到邻氨基氧-甲基-苯乙酸甲酯的盐酸盐,之后,将其与间三氟甲基苯乙酮脱去HCl和H2O,产品再与亚硝酸叔丁酯反应得到一个酮肟化合物,接着用硫酸二甲酯甲基化就可以得到最终产品。
- 1、下载文档前请自行甄别文档内容的完整性,平台不提供额外的编辑、内容补充、找答案等附加服务。
- 2、"仅部分预览"的文档,不可在线预览部分如存在完整性等问题,可反馈申请退款(可完整预览的文档不适用该条件!)。
- 3、如文档侵犯您的权益,请联系客服反馈,我们会尽快为您处理(人工客服工作时间:9:00-18:30)。
Available online at International Journal of Hydrogen Energy29(2004)569–577/locate/ijhydeneBiohydrogen production by anaerobic fermentationof food wasteSun-Kee Han∗,Hang-Sik ShinDepartment of Civil and Environmental Engineering,Korea Advanced Institute of S cience and Technology,373-1Guseong-dong,Yuseong-gu,Daejeon305-701,South KoreaAccepted15August2003AbstractThis study was conducted to improve hydrogen fermentation of food waste in a leaching-bed reactor by heat-shocked anaerobicsludge,and also to investigate the e ec t of dilution rate(D)on the production of hydrogen and metabolites in hydrogen fermentation.Among various reaction constraints a ecting the fermentation of food waste,a key factor is the adjustment of environmental conditions during the fermentation because various components of food waste have di erent characteristics of degradation.D was used as a tool to keep the optimum conditions of hydrogen fermentation.The fermentation e ciency(58%)at initial D of4:5d−1was higher than those(51.4,55.2,and53.7%)at initial D of2.1,3.6,and5:5d−1. The chemical oxygen demand(COD)removed was converted to hydrogen(10.1%),volatile fatty acids(VFA)(30.9%),and ethanol(17.0%).The butyrate/acetate(B=A)ratios were maintained over3.2in theÿrst2days.In addition,the fermentation e ciency improved from58.0%to70.8%by adjusting D from4.5to2:3d−1depending on the state of degradation.The COD removed was converted to hydrogen(19.3%),VFA(36.5%),and ethanol(15.0%).Compared to0.7–2.2with no D control, the B=A ratios were kept high(2.0–2.7)on days3–7,accompanied by the second hydrogen peak.The trend of B=A ratios was similar to the hydrogen production.D control led environmental conditions to favor hydrogen production.This meant that the fermentation e ciency was improved by the enhanced degradation of slowly degradable matters.Moreover,D control could delay the shift of predominant metabolic ow from hydrogen-and ac id-forming pathway to solvent-forming pathway.?2003International Association for Hydrogen Energy.Published by Elsevier Ltd.All rights reserved.Keywords:Hydrogen fermentation;Leaching-bed reactor;Food waste;Heat-shocked anaerobic sludge;Dilution rate control1.IntroductionThe balanced planet readily assimilates pollutants through its natural processes.However,when the planet is over-loaded with pollutants and the means by which it assimi-lates these pollutants are reduced,the planet falls away from equilibrium.The main concern about global climate change is the greenhouse e ect caused by excess carbon dioxide in the atmosphere,which relates directly with fossil fuel combustion from coal-ÿred power plants or automobiles. As a sustainable energy source,hydrogen is a promising al-ternative to fossil fuels.It is a clean and environmentally∗Corresponding author.Tel.:+82-42-869-3697;fax:+82-42-869-3610.E-mail address:skhan003@(S.-K.Han).friendly fuel,which produces water instead of greenhouse gases when combusted.Furthermore,it has a high energy yield(122kJ=g),which is about2.75times greater than that of hydrocarbon fuels,and could be directly used to produce electricity through fuel cells[1,2].Hydrogen can be generated in a number of ways,such as electrochemical processes,thermochemical processes,pho-tochemical processes,photocatalytic processes,or photo-electrochemical processes,[3,4].However,these processes do not accomplish the dual goals of waste reduction and energy production.Furthermore,these methods require electricity derived from fossil fuel combustion.For global environmental considerations,biohydrogen production from renewable organicwaste sourc es represents an impor-tant area of bioenergy production[5].Many studies have reported on the groundwork for creating renewable biohy-0360-3199/$30.00?2003International Association for Hydrogen Energy.Published by Elsevier Ltd.All rights reserved. doi:10.1016/j.ijhydene.2003.09.001570S.-K.Han,H.-S.Shin/International Journal of Hydrogen Energy29(2004)569–577drogen production systems through either photosynthesis [6–8]or fermentation[9–11].Hydrogen production by fermentative bacteria is technically simpler than by photo-synthetic bacteria.Also,the fermentation process generates hydrogen from carbohydrates obtained as refuse or waste products[5,12].As a major burden to the environment,the generation of food waste amounts to11,237tons per day in Korea, accounting for23.2%of municipal solid waste[13].Food waste is the main source of decay,odor and leachate in collection and transportation due to its high volatile solids (VS;85–95%)and moisture content(75–85%).However, as food waste has a high energy content,energy generation while reducing the waste seems ideal.Hydrogen recovery from food waste also has a potential to enhance the economic feasibility of waste treatment.The understanding of the properties of hydrogen fermentation lies in an appreciation of the response to en-vironmental changes that in uence hydrogen metabolism. Hydrogen fermentation of food waste is a ected by sev-eral factors such as pH,retention time,carbon source,etc. [14–16].Proper pH control is a key factor to improve the germination of the clostridia,as well as to initiate and op-erate a hydrogen-producing bioprocess[17].Appropriate control of retention time is also an important factor to avoid biomass washout and to increase biomass concentration in the reactor[18].The biodegradability of food waste is mainly related to carbohydrate materials,which are the main source of hydrogen production[19].Each component of food waste is degraded under the di erent environmental conditions,so that starch,cellulose,and protein should be degraded at their own optimum pHs and retention times [20].These mean that hydrogen fermentation of food waste can be improved by adjusting the environmental condi-tions.Although extensive studies were conducted on hydrogen fermentation of organicwastewaters,little is known about biohydrogen production from organic solid wastes such as food waste.This study was,therefore,conducted to maxi-mize hydrogen fermentation of food waste in a leaching-bed reactor by controlling the fermentation conditions.Dilution rate(D)was examined as a tool to keep the optimum con-ditions of hydrogen position of metabo-lites was also investigated as an indicator to evaluate the e ciency of hydrogen fermentation.2.Materials and methodsSeed microorganisms:The seed sludge was taken from an anaerobicdigester in a sewage treatment plant and boiled for15min to inactivate hydrogenotrophic bacteria and to harvest anaerobic spore-forming bacteria such as Clostridium sp.[1,21].The digester was operated at a tem-perature of35◦C and a hydraulicretention time(HRT)of 25days by feeding a mixture(1.5–2.0%VS)of primary Table1Characteristics of food wasteItem Unit Value Physical characteristicsBulk density g/l502.2 Moisture content%79.5 TS g added391.3 VS g added371.7 VS/TS0.95 Na+g/l0.84 CompositionGrains(¡10)a%TS61.1 Vegetables(¡30)a%TS29.7 Meats(¡20)a%TS9.2 Chemical characteristicsElementary analysisCarbon,C%TS51.4 Hydrogen,H%TS 6.1 Oxygen,O%TS38.9 Nitrogen,N%TS 3.5 Sulfur,S%TS0.1 C/N14.7 a Indicates the particle size in mm.sludge and waste activated sludge.The pH,alkalinity, and volatile suspended solids(VSS)concentration of the sludge were7.5,1350mg=l as CaCO3and14;600mg=l, respectively.Feedstock:Food waste,collected from a dining hall,was fed into the reactor after separating out bones and shells. Table1shows the physical and chemical characteristics of food waste.Food waste contained grains,vegetables and meats,whose composition was61.1%,29.7%and9.2%as total solids(TS).The bulk density,moisture content,VS/TS, Na+,and C/N of the waste were502:2g=l,79.5%,0.95, 0:84g=l and14.7,respectively.Experimental setup:A leaching-bed reactor with work-ing volume of3:8l was operated at35◦C,as shown in Fig.1.Dilution water was continuously delivered to the re-actor by a peristaltic pump.To collect the leachate,a perfo-rated plate and beads were installed at the lower part.The biogas production was measured using a wet gas meter. Experimental conditions:Two experiments were per-formed to improve biohydrogen production from food waste.The D s of2.1(R1),3.6(R2),4.5(R3),and5.5 (R4)d−1were kept during theÿrst experiment toÿnd opti-mal initial D,and then the obtained initial D(4:5d−1)was adjusted to4.5(R5),3.4(R6),2.3(R7),and1.2(R8)d−1 during the second experiment to maximize the fermentation e ciency.Dilution rate(D;d−1)is equal to volumetric owrate of dilution water(l/d)divided by working reactor volume(l).Experimental procedure:The seed sludge(10%v=v)was acclimated to food waste for6h,and then dilution water wasS.-K.Han,H.-S.Shin/International Journal of Hydrogen Energy29(2004)569–577571Fig.1.Schematic diagram of anaerobic leaching-bed reactor for hydrogen production.provided to the leaching-bed reactor to control environmen-tal conditions during hydrogen fermentation.The samples (25ml)of the leaching-bed reactors were collected daily during the operation period.Analytical methods:Biogas composition was ana-lyzed by a gas chromatograph(GC,GowMac series580) equipped with a thermal conductivity detector(TCD)and two columns.The contents of methane and carbon diox-ide were determined using a1:83m×3:18mm(inside diameter)stainless-steel column packed with Porapak Q (80/100mesh).Hydrogen content was measured with a 1:83m×3:18mm(inside diameter)stainless-steel col-umn packed with molecular sieve5A.The operational temperatures of injector,detector and column were kept at80◦C,90◦C and50◦C,respectively.Helium was used as a carrier gas at a owrate of40ml=min.The con-centrations of individual volatile fatty acids(VFA)were analyzed by a high-performance liquid chromatograph (LC,SpectraSYSTEM P2000)equipped with an ultravi-olet(210nm)detector and a300m×7:8mm Aminex HPX-97H column after pretreatment with a0:45 m mem-braneÿlter.H2SO4of0:005M was used as a mobile phase at a owrate of0:6ml=min.Alcohols were deter-mined by a high-performance LC(DX-600Bio-LC system) equipped with an ED50A electrochemical detector and a 250m×4mm CarboPacPA10c olumn after pretreatment with a0:45- m membraneÿlter.Deinoized water was used as the mobile phase at a owrate of0:6ml=min.The param-eters such as chemical oxygen demand(COD),VSS,and pH of the samples were measured according to Standard Methods[22].3.Results and discussion3.1.E ect of initial dilution rate(D)on biohydrogen production from food wasteFig.2illustrates the e ect of initial D on VFA,pH, and hydrogen production during hydrogen fermentation of food waste.R1showed high VFA concentration(3860mg COD/L)on theÿrst day and low pH values(4.0–4.3)in the ÿrst2days.Simple organicmatters were rapidly ac idiÿed in the initial stage[17],so that,at low D of2:1d−1,VFApH345678Time (days)01234567 CumulativeH2gas(L)VFA(mgCOD/L)Fig.2.The e ect of initial D on VFA,pH and hydrogen production during hydrogen fermentation of food waste.572S.-K.Han,H.-S.Shin/International Journal of Hydrogen Energy29(2004)569–577Table2Variation of individual VFAs,butyrate/acetate(B=A)ratio and ethanol at di erent initial D valuesD Time VFA nHBu a iHBu a HAc a HLa a HPr a nHVa a iHVa a HFo a B=A b EtOH a(d−1)(d)(mg COD=l)(%)(%)(%)(%)(%)(%)(%)(%)(mg COD=l) 2.11386010.7 3.4 6.677.50.20.60.10.9 2.19852341023.60.610.158.50.3 4.80.5 1.5 2.49203240451.10.826.1 6.5 3.6 4.4 5.9 1.6 2.011914130533.5 1.538.30.010.60.015.90.20.91635580022.4 2.037.90.114.90.722.00.10.63345660016.8 2.439.50.015.00.026.30.00.52581744016.7 2.439.60.015.00.026.30.00.516253.61310029.7 2.19.552.60.4 3.90.0 1.9 3.44412247848.20.017.022.9 1.1 6.1 1.3 3.4 2.85723143045.2 6.725.0 1.8 5.4 1.712.1 2.1 2.11014478226.1 2.630.00.013.80.026.90.6 1.01920558219.2 2.829.90.015.60.032.20.30.71000646319.3 2.530.10.014.30.033.70.20.7680740019.3 2.530.10.014.30.033.70.20.74404.51275041.2 2.511.436.40.5 4.70.8 2.5 3.83832214551.5 2.216.2 1.3 1.818.17.4 1.5 3.34653132042.9 2.120.00.08.07.318.80.9 2.2820475927.7 2.622.80.016.60.029.60.6 1.31400545120.9 1.128.60.014.10.035.10.30.8630640220.8 1.128.60.014.10.035.10.30.8315733020.8 1.128.70.014.00.035.10.30.81805.61184624.40.59.059.50.9 2.20.0 3.6 2.83372165043.10.417.325.80.8 5.0 2.2 5.2 2.5384375243.3 1.520.9 6.1 5.5 1.918.7 2.2 2.1712449425.0 3.028.00.013.20.030.10.7 1.01527529517.9 1.931.10.017.00.031.70.30.6956620119.8 1.635.30.019.70.023.60.00.6360716017.4 1.637.60.019.70.023.70.00.5240a nHBu=normal butyric acid;iHBu=iso butyric acid;HAc=acetic acid;HLa=lactic acid;HPr=propionic acid;nHVa =normal valericac id;iHVa=iso valericac id;HFo=formicac id;EtOH=ethanol.b B=A=(nHBu+iHBu)=HAc.accumulated and pH dropped.It was reported that hydro-gen evolution by Clostridium sp.was inhibited at pH4.0–5.0[23,24].Hydrogen production was,therefore,a ected by low pH and the maximum value of14:5l=d was the low-est among the reactors.On the other hand,R4indicated low VFA(1;846mg COD/L)on day1and high pH(6.2–7.5) over the operation period.At high D of5:6d−1,microbial washout might be greater than microbial growth.Thus,the low concentration of biomass in the reactor led to the de-crease of VFA production and the increase of pH.The peak (23:5l=d)of hydrogen production was the second lowest among the reactors.Although R2showed higher VFA pro-duction than R3,the maximum hydrogen production was observed in R3.At D of4:5d−1,hydrogen peak of31:0l=d was the highest among the reactors.The pH of5.5was main-tained in theÿrst2days,which was reported optimal for Clostridium sp.producing hydrogen[1,25,26].In summary,D of4:5d−1was appropriate to avoid the accumulation of excess VFA and the washout of hydrogen-producing bacte-ria in the initial stage of hydrogen fermentation.Table2lists the variation of individual VFAs,bu-tyrate/acetate(B=A)ratio and ethanol at di erent initial D s. The distribution of metabolites formed during hydrogen fermentation is often a crucial signal in assessing the e -ciency of hydrogen-producing cultures[27–29].In theÿrst 3days,all the reactors showed that hydrogen production was dominant(Fig.2)and VFA concentration was higher than ethanol.Butyrate and acetate were major components of VFAs over the whole operation,though lactate was very high on days1–2.The B=A ratios were kept higher than2.0. However,as hydrogen production ceased,VFA concen-tration became lower than ethanol on days4–7.Butyrate composition decreased drastically,while acetate,valer-ate,and propionate increased.As a result,the B=A ratiosS.-K.Han,H.-S.Shin/International Journal of Hydrogen Energy29(2004)569–577573 Table3COD balance at di erent initial D valuesDilution rate(D;d−1) 2.1 3.6 4.5 5.5 Food waste added(g COD)412.6412.6412.6412.6 COD reduction(g COD)212.3227.9239.2221.4(51.5%)(55.2%)(58.0%)(53.7%)Hydrogen production(g COD)21.229.041.524.7(5.1%)(7.0%)(10.1%)(6.0%)VFA production(g COD)94.8116.7127.7103.7(23.0%)(28.3%)(30.9%)(25.1%)Ethanol production(g COD)96.382.270.093.0(23.3%)(19.9%)(17.0%)(22.6%) COD remaining(g COD)193.4175.3167.5180.9(46.9%)(42.5%)(40.6%)(43.8%)decreased below1.3.On the other hand,when comparedwith D values,the increase of initial D from2:1d−1(R1)to4:5d−1(R3)resulted in the increase of butyrate andB=A ratio as well as in the decrease of lactate on days1–3.R3showed the highest B=A ratios of3.8–2.2in theÿrst3days.The available hydrogen during the fermentation wasdetermined using the B=A ratio[12,30].The trend of B=Aratios was similar to the hydrogen production,suggestingthat butyrate formation improved hydrogen production.The optimal B=A ratios vary in accordance with anaerobiccultures and substrate used.The highest B=A ratios in R3were comparable to2.0and5.1for Clostridium butyricumutilizing glucose and sucrose,respectively[19,31].The ad-ditional increase of initial D(5:6d−1),however,decreasedB=A ratios.It was reported that solvent production causednegative e ects on hydrogen production[1,19,32].Ethanolincreased signiÿcantly from day4when hydrogen produc-tion stopped.It indicated that heat-shocked sludge was ableto induce a metabolic ow from acid-producing pathwayto solvent-producing pathway,which was also found inbiohydrogen production from organic fraction of municipalsolid waste[1].The e ciency of hydrogen fermentation could bedescribed ase ciency of hydrogen fermentation(%)=COD pCOD o ×100;(i)where COD o is the theoretical COD of substrate(g COD) and COD p is the actual COD(e.g.,VFA,hydrogen and ethanol)produced in hydrogen fermentation of substrate at a time(g COD).The theoretical COD value(COD o)of food waste was 412:6g COD(that is,375:1g VS×1:1g COD=g VS), while the actual COD values(COD p)produced in hydrogen fermentation by day7were212.3(R1),227.9(R2),239.2 (R3)and221:4g COD(R4).Thus,the maximum e ciency, obtained by Eq.(i),was58.0%(R3)at initial D of4:5d−1, as shown in Fig.3.Table3shows that the COD removedTime (days)01234567 Efficiencyofhydrogenfermentation(%)102030405060Fig.3.The e ciencies of hydrogen fermentation of food waste at di erent initial D values.in R3was converted to hydrogen(10.1%),VFA(30.9%), and ethanol(17.0%).As1mol(22:4l)of hydrogen was equivalent to16g of COD,the COD of hydrogen was calcu-lated as:the volume(l)of H2produced×0:714g COD=LH2. The COD values of VFA and ethanol were analyzed by a LC(SpectraSYSTEM P2000)and a LC(DX-600Bio-LC system),respectively.As the tendency of COD balance is the same as that of energy balance,Table3also shows the e ciency of food waste for gaseous energy recovery as hydrogen.3.2.E ect of dilution rate(D)control on biohydrogen production from food wasteBased on the results of the preceding experiment,initial D was kept at4:5d−1in theÿrst2days.Fig.4illustrates the e ect of D control on VFA, pH,and hydrogen production during hydrogen fermenta-tion of food waste.In theÿrst2days,VFAs,pHs,and574S.-K.Han,H.-S.Shin /International Journal of Hydrogen Energy 29(2004)569–577Fig.4.The e ect of D control on VFA,pH,and hydrogen produc-tion during hydrogen fermentation of food waste.hydrogen production were maintained in the range of 2194–2836mg COD/l,5.4–5.5,and 13.0–33:9l =d,re-spectively.Although simple organic matters were rapidly degraded,excess VFA accumulation and pH drop did not occur at initial D of 4:5d −1.In R5and R6,the reduction of simple organic matters caused the decrease of VFA produc-tion and the increase of pH after 2days.Hydrogen produc-tion also decreased,as microbial proliferation was less than microbial loss by washout.However,the performance of R7was dramatically improved by reducing D from 4.5to 2:3d −1.The second VFA peak (2988mg COD/l)appeared on day 3and the pH was maintained below 6.0on days3–6.Hydrogen production was the highest among the reactors,indicating that D control resulted in the enhanced degradation of slowly degradable matters.The environmen-tal conditions became favorable to microbial growth by D shift [33].On the other hand,in R8,hydrogen production was the third lowest,even though the second VFA peak was the highest.Product inhibition caused by high VFA seemed to make the environmental conditions deteriorated [34].Accordingly,the proper D control,depending on the state of degradation,was e ective in improving the performance of hydrogen fermentation.Table 4lists the variation of individual VFAs,B=A ratio,and ethanol at various D control.At initial D of 4:5d −1(days 1–2),R5–R8showed that the concentration of VFA was higher than that of ethanol.Butyrate (40.6–51.9%)and acetate (11.4–16.7%)were the two major components of VFAs in the ÿrst 2days,though lactate composition was high on day 1.The B=A ratios were maintained higher than 3.2.The B=A ratio has frequently been used as an indica-tor for evaluating the e ectiveness of hydrogen production [31,35].High B=A ratio is favorable to hydrogen produc-tion.D was,therefore,reduced to keep high B=A ratios for enhanced hydrogen production from day pared with R5(control),the B=A ratios of R6–R8increased on days 3–7and additional hydrogen production was observed.Es-pecially,R7showed the highest B=A ratios over 2.0among the reactors on days 3–7,accompanied by the second hy-drogen peak.D control from 4.5to 2:3d −1led to the high-est hydrogen production in food waste fermentation.VFA concentration was kept higher than ethanol until day 4in R5,until day 5in R6and R8,and until day 6in R7.It in-dicated that D control could delay the shift of predominant metabolic ow from hydrogen-and ac id-forming pathway to solvent-forming pathway.Fig.5shows the fermentation e ciencies estimated us-ing Eq.(i ).The theoretical COD (COD o )of food waste was 412:4g COD (that is,374:9g VS ×1:1g COD/g VS),and the actual COD (COD p )produced in hydrogen fermenta-tion on day 7were 243.6(R5),271.1(R6),292.1(R7),and 260:8g COD (R8),respectively.Thus,the maximum fer-mentation e ciency,obtained by Eq.(i ),was 70.8%(R7)at D control of 4.5–2:3d −1.Table 5shows that the COD removed in R7was converted to hydrogen (19.3%),VFA (36.5%),and ethanol (15.0%).This means that the environ-mental constraints of hydrogen fermentation could be gov-erned through the proper D control depending on the state of degradation.4.ConclusionsSuccessful operation of a reactor can be accomplished when it is operated at proper D depending on the state of degradation.Operation at high D leads to the washout of biomass in the reactor,while operation at low D leads to product inhibition due to the accumulation of excess VFA.S.-K.Han,H.-S.Shin/International Journal of Hydrogen Energy29(2004)569–577575 Table4Variation of individual VFAs,butyrate/acetate(B=A)ratio and ethanol at various D controlD Time VFA nHBu a iHBu a HAc a HLa a HPr a nHVa a iHVa a HFo a B=A b EtOH a(d−1)(d)(mg COD=l)(%)(%)(%)(%)(%)(%)(%)(%)(mg COD=l) 4.5–4.51281340.6 2.111.438.20.4 4.20.6 2.5 3.73832219450.9 2.016.5 1.5 2.119.3 6.2 1.5 3.24653135042.8 2.120.20.08.37.518.20.9 2.2820477628.1 2.624.10.016.90.027.70.6 1.31400546121.1 1.030.10.014.20.033.30.30.7630641121.1 1.030.10.014.20.033.30.30.7315733821.1 1.030.10.014.20.033.30.30.71804.5–3.41281741.2 2.311.736.40.5 4.50.8 2.6 3.73742220550.7 2.216.7 1.6 2.219.1 6.0 1.5 3.24733459044.3 2.120.60.07.7 5.918.80.7 2.317044340038.5 1.224.80.012.50.022.80.3 1.628805204035.9 2.524.80.012.40.024.30.1 1.548006144534.9 2.525.80.012.40.024.30.1 1.425207102034.9 2.525.80.012.40.024.30.1 1.415604.5–2.31283642.5 2.411.934.90.5 4.40.7 2.7 3.83802222250.9 2.316.2 1.3 2.519.7 5.7 1.4 3.36803298848.1 2.018.80.08.2 4.716.2 2.1 2.711224161941.2 3.620.90.013.70.020.40.2 2.112675108339.3 3.821.90.011.20.023.70.1 2.02840673839.3 3.821.90.011.20.023.70.1 2.01083741139.3 3.821.90.011.20.023.70.1 2.06094.5–1.21282442.3 2.511.735.10.4 4.30.8 2.9 3.84052222851.9 2.216.2 1.4 2.118.6 6.0 1.6 3.35033205346.0 1.919.70.07.0 6.818.30.3 2.47964108838.8 2.022.90.015.50.020.70.1 1.8855578128.4 2.627.60.013.80.027.50.1 1.11721663828.4 2.627.60.013.80.027.50.1 1.1741757228.4 2.627.60.013.80.027.50.1 1.1399a nHBu=normal butyric acid;iHBu=iso butyric acid;HAc=acetic acid;HLa=lactic acid;HPr=propionic acid;nHVa =normal valericac id;iHVa=iso valericac id;HFo=formicac id;EtOH=ethanol.b B=A=(nHBu+iHBu)=HAc.Fig.5.The e ciencies of hydrogen fermentation of food waste at various D control.These appear to limit the production of hydrogen to reach a higher level.Firstly,the leaching-bed reactor treating food waste was operated at various D s toÿnd the optimal initial D for hydrogen pared with other D values, initial D of4:5d−1resulted in higher B=A ratios during the fermentation.The trend of B=A ratios was similar to the hy-drogen production,suggesting that butyrate formation fa-vored hydrogen production.Operation at initial D of4:5d−1 led to higher fermentation e ciency(58%)than those(51.5, 55.3and53.7%)at2.1,3.6,and5:5d−1.The COD re-moved was converted to hydrogen(10.1%),VFA(30.9%), and ethanol(17.0%).Optimum initial D was,therefore, 4:5d−1for biohydrogen production by heat-shocked anaer-obicsludge.Secondly,based on the preceding experimental result,op-eration by D control was performed to improve the fermen-tation e ciency.Without D control,the reduction of simple organic matters causes the decrease of VFA production and576S.-K.Han,H.-S.Shin/International Journal of Hydrogen Energy29(2004)569–577Table5COD balance at various D controlDilution rate(D;d−1) 4.5–4.5 4.5–3.4 4.5–2.3 4.5–1.2 Food waste added(g COD)412.4412.4412.4412.4 COD reduction(g COD)243.6271.1292.1260.8(59.1%)(65.7%)(70.8%)(63.2%)Hydrogen production(g COD)42.960.179.453.4(10.4%)(14.6%)(19.3%)(12.9%)VFA production(g COD)130.6139.9150.7131.7(31.7%)(33.9%)(36.5%)(31.9%)Ethanol production(g COD)70.171.162.075.7(17.0%)(17.2%)(15.0%)(18.4%) COD remaining(g COD)155.2130.4108.4143.3(37.6%)(31.6%)(26.3%)(34.7%)the increase of pH.Hydrogen production also decreases,as microbial proliferation becomes less than microbial loss by washout.However,the reactor performance was dramati-cally improved at D control of4.5–2:3d−1.It showed a higher B=A ratio than any other reactor on days4–7.The second hydrogen peak appeared on day4,resulting in the highest fermentation e ciency(70.8%)among the reactors. It was caused by the enhanced degradation of slowly degrad-able matters.The COD removed was converted to hydro-gen(19.3%),VFA(36.5%),and ethanol(15.0%).Proper D control could lead the environmental conditions favor-able to microbial growth and delay the shift of predominant metabolic ow from hydrogen-and ac id-forming pathway to solvent-forming pathway.Therefore,the strategy using D control,depending on the state of degradation,was e ective in improving the e ciency of hydrogen fermentation. Anaerobicbac teria use organicsubstanc es as the sole source of electrons and energy,converting them into hydro-gen.The reactions involved in hydrogen production(Eq.(ii) and Eq.(iii))are rapid and do not require solar radiation, making them useful for treating large quantities of wastew-ater by using a large fermenter.Since they cannot utilize light energy,the decomposition of organic substrates is in-complete and organic acids remain.Glucose+2H2O→2acetate+2CO2+4H2G=−184:2kJ(ii) Glucose→butyrate+2CO2+2H2G=−257:1kJ(iii) Nevertheless,these reactions are still suitable as an initial step of hydrogen production from waste,which is followed by methanogenesis.A two-stage process is a rational conÿg-uration because it provides the preferred environments for acidogenic hydrogenesis and methanogenesis in two sepa-rate spaces.The data obtained in this study is,therefore,valuable to the development of a two-stage process treating organicsolid waste.AcknowledgementsThis research was supported by a Grant(M1-0203-00-0063)from the Korea Ministry of Science and Technology, through the National Research Laboratory Program.References[1]Lay JJ,Lee YJ,Noike T.Feasibility of biological hydrogenproduction from organic fraction of municipal solid waste.Water Res1999;33:2579–86.[2]Mizuno O,Dinsdale R,Hawkes FR,Hawkes DL,NoikeT.Enhancement of hydrogen production from glucose by nitrogen gas sparging.Bioresource Technol2000;73:59–65.[3]Momirlan M,Veziroglu T.Recent directions of worldhydrogen production.Renewable Sustainable Energy Rev 1999;3:219–31.[4]Momirlan M,Veziroglu T.Current status of hydrogen energy.Renewable Sustainable Energy Rev2002;6:141–79.[5]Benemann J.Hydrogen biotechnology:progress andprospects.Nat Biotechnol1996;14:1101–3.[6]Kumar A,Jain SR,Sharma CB,Joshi AP,Kalia VC.IncreasedH2production by immobilized microorganisms.World J Microbiol Biotechnol1995;11:156–9.[7]Kataoka N,Miya A,Kiriyama K.Studies on hydrogenproduction by continuous culture system of hydrogen producing anaerobic bacteria.Water Sci Technol1997;36: 41–7.[8]Yokoi H,Maeda Y,Hirose J,Hayashi S,Takasaki Y.H2production by immobilized cells of Clostridium butyricum on porous glass beads.Biotechnol Tech1997;11:431–3. [9]Fascetti E,Todini O.Rhodobacter sphaeroides RV cultivationand hydrogen production in a one-and two-stage chemostat.Appl Microbiol Biotechnol1995;44:300–5.[10]Markov T,Miura Y,Fukatsu K,Miyasaka H,IkutaY,Matsumoto H,Hamasaki A,Shioji N,Mizoguchi T,。