IHTC16-21954 电子冷却微通道气体节流的实验研究
NACA0021和NACA4822翼型肋通道中环境空气流动传热特性的实验研究
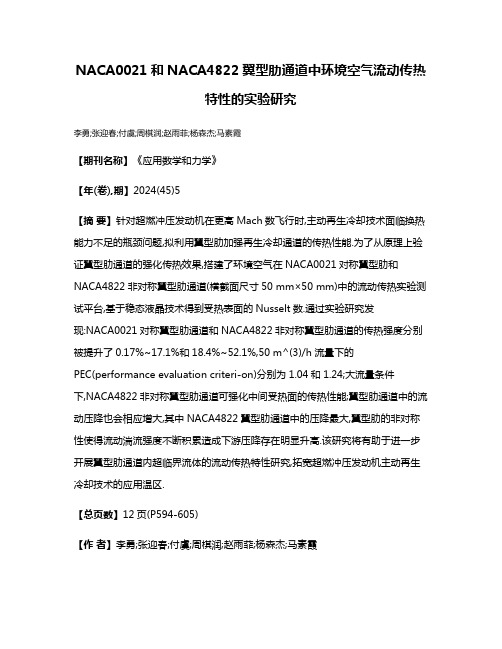
NACA0021和NACA4822翼型肋通道中环境空气流动传热特性的实验研究李勇;张迎春;付虞;周棋润;赵雨菲;杨森杰;马素霞【期刊名称】《应用数学和力学》【年(卷),期】2024(45)5【摘要】针对超燃冲压发动机在更高Mach数飞行时,主动再生冷却技术面临换热能力不足的瓶颈问题,拟利用翼型肋加强再生冷却通道的传热性能.为了从原理上验证翼型肋通道的强化传热效果,搭建了环境空气在NACA0021对称翼型肋和NACA4822非对称翼型肋通道(横截面尺寸50 mm×50 mm)中的流动传热实验测试平台,基于稳态液晶技术得到受热表面的Nusselt数.通过实验研究发现:NACA0021对称翼型肋通道和NACA4822非对称翼型肋通道的传热强度分别被提升了0.17%~17.1%和18.4%~52.1%,50 m^(3)/h流量下的PEC(performance evaluation criteri-on)分别为1.04和1.24;大流量条件下,NACA4822非对称翼型肋通道可强化中间受热面的传热性能;翼型肋通道中的流动压降也会相应增大,其中NACA4822翼型肋通道中的压降最大,翼型肋的非对称性使得流动湍流强度不断积累造成下游压降存在明显升高.该研究将有助于进一步开展翼型肋通道内超临界流体的流动传热特性研究,拓宽超燃冲压发动机主动再生冷却技术的应用温区.【总页数】12页(P594-605)【作者】李勇;张迎春;付虞;周棋润;赵雨菲;杨森杰;马素霞【作者单位】太原理工大学电气与动力工程学院;煤电清洁控制教育部重点实验室;中北大学能源与动力工程学院【正文语种】中文【中图分类】O35;V19【相关文献】1.再生冷却弧肋通道跨临界流动与传热特性数值研究2.肋片通道内圆柱扰流的流动与传热特性研究3.交错内肋微通道的流动和传热特性研究4.肋槽组合式微通道流动与传热特性研究5.含肋柱微通道换热器内纳米氧化铝流体流动传热特性研究因版权原因,仅展示原文概要,查看原文内容请购买。
微通道液-液两相流动特性实验研究

第12期 收稿日期:2020-04-22作者简介:张井志(1989—),山东枣庄人,助理研究员,主要从事多相流与强化传热技术。
微通道液-液两相流动特性实验研究张井志,李慧玲,雷 丽(山东大学能源与动力工程学院,山东济南 250061)摘要:利用高速摄像机与Canny算法,以硅油为离散相,含0.5%SDS的蒸馏水为连续相,研究了凹穴型微通道内液-液两相流动特性。
结果表明,直通道内观察弹状流、过渡流、滴状流3种流型。
随着毛细数的增大,液滴形成机理由挤压机制向剪切机制转变,液滴速度逐渐增大,液滴长度逐渐减小。
随着连续相流量的增加,液滴形成时间逐渐减小,且挤压机制生成液滴的时间大于剪切机制。
凹穴结构减弱了壁面对液滴的限制,液滴速度降低,T型交汇处压差降低,相同工况下的液滴尺寸大于对冲T型微通道的液滴尺寸。
关键词:微通道;两相流;液滴;毛细数;通道结构中图分类号:O363.1 文献标识码:A 文章编号:1008-021X(2020)12-0029-03 微化工是一项跨学科的高新技术,集合了化工、机械、电子、物理和材料等领域的研究成果。
由于装置小型化,该技术具有体系反应的效率高、反应时间短、反应环境稳定、安全性高以及容易控制流态等优点[1-3]。
根据微通道中工质的不同,主要有气-液[4-6]与液-液[7-10]两相流。
液-液两相的流动受微通道尺寸空间的限制,离散相尺寸较为均一,相界面积比高传统反应器1~3个数量级,故从液-液流动方面看,通过调控微通道流型能有效改善反应过程[11-14]。
T型微通道结构简单,生成的微液滴分散性好、大小均一,因此应用广泛。
韩宇[15]对液滴生成过程受力分析,指出气泡生成基于挤压模式、过渡模式、剪切模式。
王晓军等[16]、季喜燕[17]通过模拟T型微通道液-液两相流,分析得到通道下游远离通道壁面的离散相速度远大于T型结口近壁面离散相速度。
Dessimoz等[18]利用实验方法观察了不同当量直径的方形微通道内液-液两相流流型。
微通道换热器的R404A制冷系统性能研究的开题报告

微通道换热器的R404A制冷系统性能研究的开题报告一、选题背景和意义随着经济的发展和社会进步,制冷设备已在生产生活中广泛应用,同时,采用清洁能源、减少环境污染的要求也越来越强烈。
微通道换热器是一种新型的高效换热器,其优点在于占用空间小、传热效果好、制造成本低、易于维护等。
因此,研究微通道换热器在制冷系统中的应用,对于提高制冷系统的效率、降低制冷成本、减少环境污染具有重要意义。
本论文将以R404A制冷系统为研究对象,探究在微通道换热器中的性能研究、传热性能及其对制冷系统性能的影响,为高效的制冷系统的研究提供理论和实践基础。
二、研究内容和方案本次研究将进行如下内容:1.对微通道换热器的结构、性能进行分析,探究微通道换热器的传热机理。
2.搭建R404A制冷系统实验平台,研究R404A制冷系统在常规换热器和微通道换热器下的性能差异。
3.对实验结果进行数据分析,并综合分析微通道换热器的传热性能及其对制冷系统性能的影响。
4.基于实验结果,提出改善微通道换热器性能的技术方法和措施,以期实现高效率、低成本的制冷系统。
三、可行性分析1. 实验平台搭建:本研究使用现有设备进行组装和改装,具有良好的可操作性和可实现性。
2. 数据分析:本研究将采用科学的、系统的数据分析方法,进行数据处理和分析,具备足够的可靠性。
3. 结果的实用性:本研究旨在探究微通道换热器在制冷系统中的应用及其传热性能,得到的结果可供制冷系统设计、制造和使用阶段参考,具有实用性和推广价值。
四、预期成果1. 详细分析微通道换热器的结构、传热机理。
2. 对比微通道换热器和传统换热器在制冷系统中的性能差异,揭示微通道换热器的优势和劣势。
3. 分析微通道换热器对制冷系统整体性能的影响,为制冷系统的设计和改进提供有力依据。
4. 提出改善微通道换热器性能的技术方法和措施,为高效率、低成本的制冷系统提供理论和实践支持。
五、进度安排论文研究的时间进度预计为一个学期,具体进度安排如下:第一周:文献资料检索和阅读,研究现状分析。
液氦温区小型节流制冷机发展现状及趋势
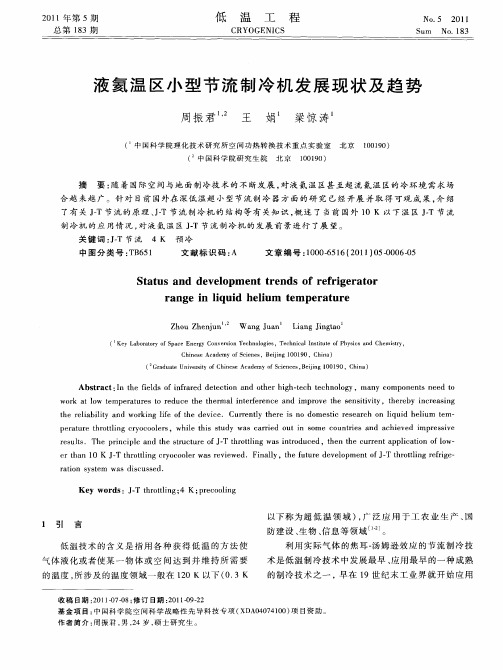
关 键 词 :. 节 流 JT 4 K 预 冷
中 图 分 类 号 :B 5 T 61
文 献 标 识 码 : A
文 章 编 号 :0 06 1 ( 0 1 0 —0 60 1 0 —5 6 2 1 )50 0 5
Absr t I he fed fifa e e e t n a t e ih—e h tc n lg tac : n t l s o nr r d d tc i nd o h r h g tc e h o o y,m a y c mp n n sn e O i o n o o e t e d t
温 度 降低 至 2点 , 12表示 。接 着 高压 气 体经 过 节 用 . 流 阀产 生 制冷 后压 力 降低 即 23过程 , — 回流 的气体 在
热 交换 器与 高温 气体 热交 换温 度升 高 , 想状 态下 温 理
表 1 不 同气 体 的最 高转 化温 度
Tabl Top t a i i e pe at e o f e e as e1 r ns ton t m r ur f di r nt g
wo k a o tmpe au e o r d c h h r a n ef r n e a d i r v h e i vt t e e n r a i g r tlw e r t r s t e u e t e t e m li tre e c n mp o e t e s nst i i y, h r by i c e sn t e r la iiy a d wo k n ie o h v c . Cu r n l h r s n o si e e r h o i u d h lu t m— h eib lt n r i g lf ft e de ie re ty t e e i o d me tc r s a c n lq i ei m e p r t r h o tig c y c oe s,wh l hi t d s c rid o t i o o ntis a d a hiv d i r s ie e au e t r tl r o o lr n i t s su y wa a re u n s me c u re n c e e mp e sv e
浅谈微通道换热器的发展
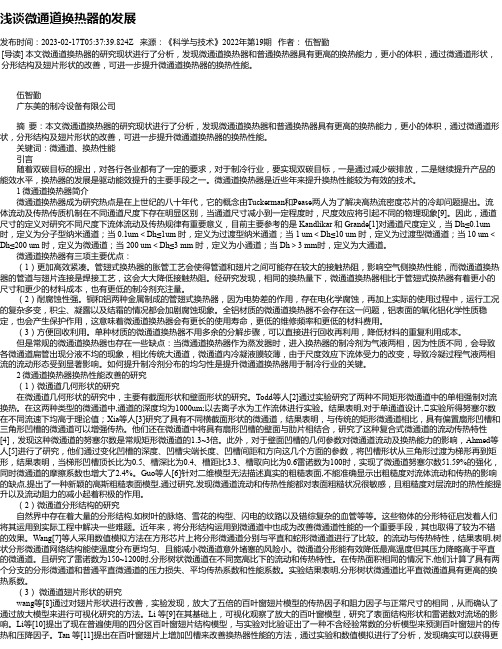
微通道内单相及气液两相流动换热数值模拟研究进展综述
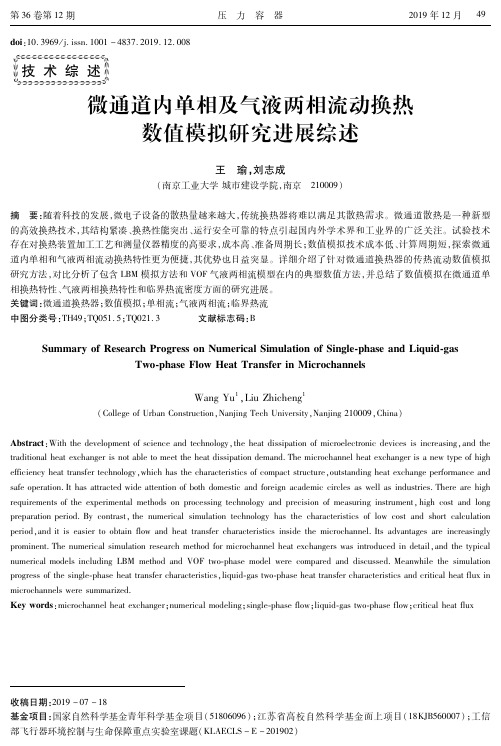
0 引言
微通道换热器的工程背景来源于 20世纪 80 年代高密度电子器件的冷却和 20世纪 90年代出 现的微电子机械系统的传热问题。随着能源问题 的日渐突显,各国的经济发展与微小器件的发展 息息相关,换热设备在满足热交换要求的前提下, 需要向缩小体积的方向优化,以节约更多空间和 能源[1]。随着微型换热设备的出现和普及,微尺 度传热问题也成为换热器试验和数值模拟研究的 重点。Tuckerman等[2]提出了如图 1所示的微通 道换热器,通过多个细微通道内的介质流动带走 电子芯片积聚的热量,成功地解决了随着科技发 展、芯片集成度越来越高带来的高热流密度散热 问题。孙淑风等[3]研究了液氮在尺寸为 0.55~ 1.5mm的微通道中流动沸腾的传热效果,发现狭 窄通道的强制对流沸腾换热对沸腾换热具有强化 作用,其中,液氮在狭窄通道形状为弦月型的传热 系数是常规尺寸管道的 3~5倍,随着狭窄的间隙 尺寸的减少,换热系数也得到提高。综上所述,微 通道换热因其体积小、换热效率高、耐压性能强等 特点被认为是最有发展前景的高热流密度散热技 术之一。
微通道冷凝器空气侧性能的实验研究
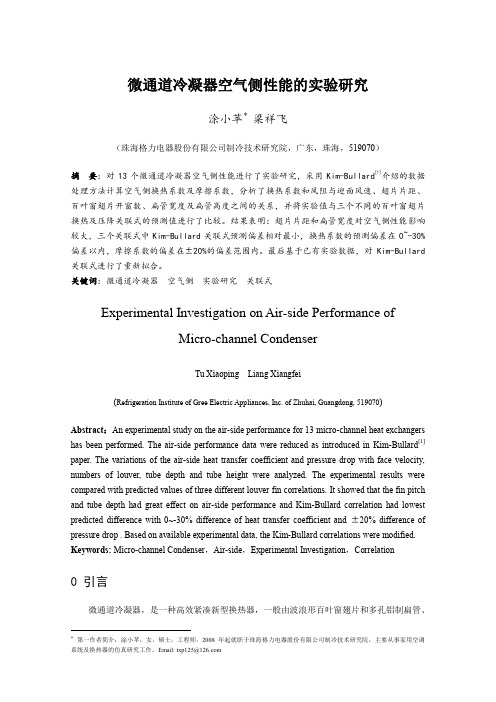
100
18*2-10-1.2-10 18*2-10-1.2-12
风阻/Pa
80 60 40 20
风阻/Pa
16*1.8-16-1.2-10 16*1.8-18-1.2-10 16*1.8-18-1.2-12 16*1.8-16-1.2-12
3 实验结果及分析
前 7 个样件水流量较小,水侧流动换热情况不能用常用的紊流或者包含过渡区的换热关 联式计算,换热性能用换热量来衡量。后 6 个样件的实验数据依据 Kim-Bullard[1]介绍的方法 进行处理,换热性能用换热系数来衡量。 图 2~5 分别示出了百叶窗翅片不同结构参数下空气侧换热与压降的变化情况,图中各样 件名称按扁管宽度*扁管高度-孔数-翅片片距-开窗数表示,例如 16*1.8-16-1.2-10 表示扁管宽 度为 16mm,扁管高度为 1.8mm,16 个孔,翅片片距 1.2mm,翅片开窗数为 10 的微通道换热
从图 2 可以看出,随着翅片片距的增加,空气侧换热能力下降,风阻也下降,且高迎面 风速时风阻下降幅度更大,这与其他学者的研究结论是一致的。因此,当迎面风速较高时, 翅片片距不宜选得太大。 3.2 开窗数的影响 表 2 给出了迎面风速 1.4m/s 时,5#(18*2-10-1.2-10)和 6#(18*2-10-1.2-12)两个样件对 应的换热量和风阻值及相对偏差。
(3)
式(3)中 Kc 和 Ke 是空气流入和流出换热器的压力损失系数。Kays—London(1984)指 出,如果换热器的翅片形式复杂,比如百叶窗翅片(Louver fin) ,当流体流入换热器后,由于 百叶窗翅片的充分扰动,流体流动为紊流,此时以 ReDh→∞来估算 Kc 和 Ke 值,此时各种翅片 形式的换热器 Kc 和 Ke 值都是相同的,只与流道收缩比有关,具体取值按照 Kays—London (1984)的《紧凑式换热器》一书中相关的图表获得。
微通道冷凝器空气侧性能的实验研究
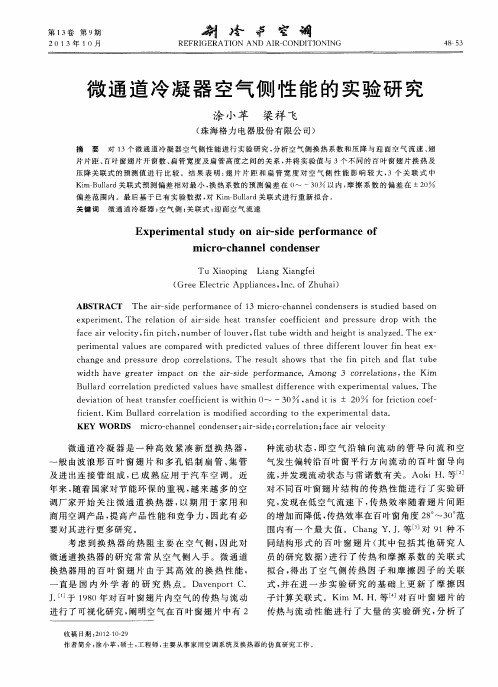
p e r i me n t a 1 v a l u e s a r e c o mp a r e d wi t h p r e d i c t e d v a l u e s o f t hr e e d i f f e r e nt l ou v e r f i n h e a t e x — c ha n ge a n d pr e s s u r e dr o p c o r r e l a t i o ns .Th e r e s u l t s h ows t ha t t he f i n pi t c h a n d f l a t t u be
( Gr e e El e c t r i c App l i a n c e s, I n c . o f Zh uh a i )
ABS TRACT The a i r — s i de p e r f o r ma nc e o f 1 3 mi c r o — c h a nn e 1 c o nd e n s e r s i S s t u di e d b a s e d o n e xp e r i me n t . T he r e l a t i o n o f a i r — s i d e he a t t r a n s f e r c o e f f i c i e n t a nd p r e s s ur e dr o p w i t h t h e f a c e a i r v e l o c i t y, f i n pi t c h, nu m be r of l ou v e r, f l a t t ub e wi d t h a nd he i g ht i s a n a l y z e r o — e ha n ne 1 c on de ns e r ; a i r — s i d e: c o r r e l a t i o n; f a c e a i r v e l o c i t y
- 1、下载文档前请自行甄别文档内容的完整性,平台不提供额外的编辑、内容补充、找答案等附加服务。
- 2、"仅部分预览"的文档,不可在线预览部分如存在完整性等问题,可反馈申请退款(可完整预览的文档不适用该条件!)。
- 3、如文档侵犯您的权益,请联系客服反馈,我们会尽快为您处理(人工客服工作时间:9:00-18:30)。
Proceedings of the 16th International Heat Transfer Conference, IHTC-16August 10-15, 2018, Beijing, ChinaIHTC16-21954*Corresponding Author: jhweng@EXPERIMENTAL INVESTIGATION OF GAS THROTTLING INJianhua Weng 1*1, Geng Hui 2,Xiaoyu Cui 2, Tongyun Zhang 1, Tenghui Liu 11Shanghai University of Electric Power, 2103 Ping Liang Rd. Yangpu District, Shanghai 200090, China 2University of Shanghai for Science and Technology, 516 Jun Gong Rd. Yangpu District, Shanghai 200093,ChinaABSTRACTThis experimental investigation was intended to evaluate the capability of micro-coolers designed with microchannel for electronic cooling. Two prototypes of micro-cooler were fabricated for the experiments. Both of them had a counter-flow heat exchanger, which consisted of 7 layers of microchannel. Each layer had 6 microchannels. The throttling process was carried out with gas flowing through microchannels of smaller size. The two prototypes of micro-cooler had similar configuration but different cross-sectional area of microchannels for the heat exchanger and the throttle. In the experiments the temperature of several locations along the surfaces of the micro-coolers was measured. Experiments with different gas inlet pressure and temperature were carried out and experimental results were obtained. It showed that for the throttling process the higher the inlet gas pressure, the larger the temperature drop. And for inlet temperature, the lower the inlet gas temperature, the larger the temperature drop. From the experiment results, the cooled gas after throttling could be used for cooling electronic devices with high-heat-flux. It can be considered as one of the prospective electronic cooling methods for further study.KEY WORDS: Electronic cooling, Heat dissipation, Throttling process, Microchannels1. INTRODUCTIONAs heat flux from electronic devices continues to rise, heat dissipation for these devices remains a great challenge. New technologies have been proposed to remove the heat of high-heat-flux, such as microchannels, spray cooling, etc. [1]. The Joule-Thomson(J-T) cryocooler has been used for cooling infrared sensors on satellites and cryosurgery for some time. The J-T cryocooler usually consists of a counter-flow heat exchanger, a throttling (capillary) device and a refrigerating cavity[2]. The J-T cryocooler can be an open cycle or a closed cycle[3]. The open cycle cryocooler uses a gas reservoir, and it has the advantages of simplicity, small size, low weight, and absence from mechanical noise or vibration[4].The working fluid used in J-T crycoolers includes argon, CO 2, N 2, etc.. The closed cycle cryocooler has a compressor, and mixed refrigerants are considered to be used as working fluid[5]. Derking et al.[6] investigated a cooling system using a gas mixture of methane, ethane and isobutene, with cold end temperature reaching at 150K and cooling power of 46mW. Ardhapurkar et al.[7]used mixture of N 2 and CO 2 as working fluid in a J-T cryocooler. Naer et al.[8] applied hydrocarbon mixtures in a closed micro cooler system for the temperature range of -73 to -183℃. In commercial application, Hampson-type J-T cryocooler has been widely adopted and the conventional one has a large mandrel at the center of the cryocooler. Since Tuckerman and Pease [9] used microchannels to remove heat with high-heat-flux, heat transfer and fluid flow in microchannles has been studied extensively. Colgan et al.[10] designed a Si microchannel cooler for cooling high power chips. The test showed the designed cooler demonstrated the capability of heat removal with heat flux greater than 300W/cm 2. Wei et al. [11]fabricated a stacked microchannel heat sink with two layers of microchannels, and the total thermal resistance could be as low as0.09℃/(W/cm 2). To minimize the size of cryocoolers, Little[12] introduced microchannels into these small cooling devices. Besides small size, these cryocoolers also have the features of fast response and low noise. They could easily be integrated into other instruments. Lerou et al.[13] designed 8 types of cooler and fabricated 14 prototypes. One of the configurations had the microchannel with 2.2mm in width and 0.5mm in depth, and with a net cooling power of 20mW at about 100K. Hatwar et at.[14] fabricated an open cycle miniature J-T refrigerator with a single microchannel, using low temperature co-fired ceramic technique. The dimension of the microchannel was 1mm in width and 0.25mm in depth. Using N 2 gas as working fluid, temperature drops of 22 to 46K were achieved with the inlet gas pressure ranging from 40 to 80bar. Wang et al.[15] fabricated and tested a polymer-based J-T micro cryogenic cooler. It contained a polymer heat exchanger and a silicon/glass J-T valve.This paper presents the experimental results of two J-T micro-coolers using microchannel both for the heat exchanger and throttling device. And gas of CO 2 and argon were used as working fluid.2. GAS FLOWING AND THROTTLING IN MICROCHANNELS2.1 Microchannels For gas flowing in microchannels, four flow regimes can be defined by Knudsen number: LKn λ= (1)Based on the value of Kn, the four flow regimes are continuum flow(Kn<10-3), slip flow(10-3<Kn<10-1), transition flow(10-1<Kn<10) and free molecular flow(Kn>10), respectively[16]. For continuum flow, the flow mathematically still can be described by Navier-Stokes equations with classical no-slip boundary conditions. For example, gas of CO 2 with pressure of 1atm and temperature of 300K flowing in a channel with cross section of 0.3mm by 0.4mm, the Kn is calculated to be less than 9×10-5, and the flow can be considered as continuum flow.2.2 Gas Throttling When a real gas passes through a capillary tube or a valve, its pressure decreases. The enthalpy usually is considered as approximately constant for such a throttling process. After throttling, the gas temperature might decrease, remain unchanged, or even increase, depending on the value of J-T coefficient[17]: h p ⎪⎪⎭⎫ ⎝⎛∂∂=T JT μ (2) At room temperature, gases such as CO 2, N 2 and argon cool down during the throttling process, as their J-T coefficients are positive. Situations are similar when gas flowing through a microchannel. Pressure of the gas will decrease and its temperature will drop if its J-T coefficient is positive.3. EXPERIMENTAL SETUP AND TEST PROTOTYPES3.1 Experimental Setup The experimental setup was an open system as shown in Fig. 1. Gas of CO 2 or argon came from a cylinder tank, and its flow rate was measured by a flowmeter. The pressure of the gas was regulated by a valve close to the outlet of the tank. The test prototype was enclosed by a chamber with a vacuum pump withdrawing the air inside the chamber, so the prototype was thermally isolated with the environment. After the throttling process in the microchannel of the test prototype, the low temperature gas was eventually discharged to the environment through a pipe. Pressure and temperature at inlet and outlet of the prototype as well as temperature of several points along the surface of the prototype (see Fig.2) were measured. All the measured data were collected by a data acquisition system. The range and accuracy of instruments used in the experiment are listed in Table 1.3.2 Test Prototypes The prototype consisted of 7 layers for high and low pressure gas. Fig.3a shows the plate for high pressure gas of prototype A, which had 6 microchannels for the heat exchanger and one microchannel for the throttling process. Fig.3b shows the plate for low pressure gas of prototype A. The plates were made of stainless steel. For prototype A, the cross-section dimension of microchannel for thethe top and bottom layer, see Fig.4), while that for the throttling microchannel was 0.1mm by 0.1mm.1-Gas Cylinder 2-Inlet Valve 3-Gas Filter and Drier 4-Mass Flowmeter5-High Pressure Transducer 6-Data Acquisition System 7-Prototype and Vacuum Chamber8-Low Pressure Transducer 9-Vacuum Gauge 10-Vacuum Pump 11-Outlet ValveFig. 1 Schematic of the experimental setupFig. 2a Temperature measurement points on the surface of prototype AFig. 2b Temperature measurement points on the surface of prototype BTable 1 Measurement range and accuracy of instrumentsMeasurement Instrument Range AccuracyThermocouple for prototype surface measurement-73~215℃ ±0.2℃ Thermocouple for gas measurement at inlet andoutlet-200-400℃ ±0.1℃ High Pressure Transducer 0-10MPa 0.2 GradeLow Pressure Transducer 0-10kPa 0.2 GradeMass Flowmeter 0-300SLPM 1%The length of throttling microchannel was 40mm. There was one microchannel for throttling on each layerfor high pressure gas. For prototype B, the cross-section dimension of microchannel for the heat exchangerbottom layer), and that for the throttling channel was 0.15mm by 0.1mm. The length of throttling microchannel also was 40mm. There were 6 microchannels for the throttling on each layer for high pressure gas. Layer arrangement at the section of heat exchanger for both prototype A and prototype B is shown in Fig.4. Except for the top and bottom layer, each layer consisted of 2 plates, and microchannels were formed when two plates for high pressure or two for low pressure were mounted together. An electric heater was mounted at the cold end of the prototype, as shown in Fig.5.1-Inlet 2-Entrance Section 3-Outlet 4-Microchannels for heat exchanger5-Microchannel for throttling 6-ChamberFig.3a Microchannels for heat exchanger and microchannel for throttling process, high pressure, prototype AFig.3b Microchannles for heat exchanger, low pressure, prototype AFig.4 Layer arrangement at the section of heat exchanger, for both prototype A and prototype BFig.5 Location of the heater4. RESULTS AND DISCUSSIONSExperiments of gas flowing through both prototype A and B were carried out. Fig.6-9 show the measured temperature along the prototype surface for gas of CO2. Fig.6-7 show at the beginning all the temperature decreased but a few minutes later the temperature gradually reached steady values. Fig.8-9 indicate the lowest temperature of the measurement points was located at the end of the prototypes, which was just after the throttling microchannels. The thermocouple (TC) no. 2 to 5 for both prototypes were located on the surface ofheat exchanger. The gas flowing through microchannels before throttling was cooled by the low temperature gas after the throttling process, which was flowing in the opposite direction, and the walls of microchannels were cooled as well. Besides, the temperature decrease of TC no.2 to 5 was also partly caused by the heat conduction along the microchannel wall as the temperature at the end of the prototypes was low.Fig.6 Temperature varies with time with inlet gas pressure 3MPa and temperature 12℃,Prototype A, gas of CO2Fig.7 Temperature varies with time with inlet gas pressure 1.4MPa and temperature 12℃,Prototype B, gas of CO2Fig.8 Readings of thermocouples on surface of prototype A, steady state, gas of CO2Fig.9 Readings of thermocouples on surface of prototype B, steady state, gas of CO2Among the measurement points, the temperature of TC no. 11 for prototype A was the lowest temperature. The temperature of TC11 for different inlet conditions are listed in Table 2. It shows at the same inlet temperature, the temperature of TC11 with higher gas inlet pressure was lower than that with lower gas inlet pressure. For example, with CO2inlet gas temperature at 12℃, the reading of TC11 was -45.03℃for 4.2MPa inlet gas pressure at steady state, while that of TC11 was -26.68℃ for 3MPa inlet gas pressure. It also shows at the same inlet pressure, the temperature of TC11 with lower inlet gas temperature was lower than that with higher inlet gas temperature, e.g. with CO2 inlet gas pressure at 3MPa, the reading of TC11 was -26.68℃ for 12℃ inlet gas temperature at steady state, while that of TC11 was -13.65℃ for 21℃ inlet gas temperature.Table 2 Readings of thermocouple no.11 for prototype A at different inlet temperature and pressure, gas of CO2Inlet Temperature/℃Inlet Pressure/MPa Reading of TC11/℃12 3 -26.684.2 -45.0321 3 -13.654.2 -26.09For prototype B, the lowest temperature among the measurement points was located at the same place as prototype A. However, the TC no. for this point for prototype B experiment was no. 9, instead of no. 11. Table 3 shows the readings of TC9 at different CO2 inlet gas pressure but same inlet gas temperature. The results were the same as that of prototype A, which was that at the same inlet temperature the lowest temperature among measurement points with higher inlet gas pressure was lower than that with lower inlet gas pressure. This also was true for gas of argon as shown in Table 4. However, as mentioned above, the prototype B had larger cross section area for gas throttling comparing with prototype A, which resulted in larger flow rate for prototype B. Therefore, for prototype B with CO2 gas at inlet pressure of 1.4MPa, the lowest measured temperature could be as low as -33.46℃, while for prototype A at even higher inlet pressure of 3MPa that was -26.68℃ at same inlet gas temperature, as listed in Table 2.Table 3 Readings of thermocouple no.9 for prototype B at different inlet pressure, gas of CO2 Inlet Temperature/℃Reading of TC9/℃12 1 -14.461.4 -33.46Table 4 Readings of thermocouple no.9 for prototype B at different inlet pressure, gas of argon Inlet Temperature/℃Inlet Pressure/MPa Reading of TC9/℃16 3 -17.875.3 -43.957.3 -51.26When the cold end of prototype B was heated by an electric heater (see Fig. 5), the temperature reading of TC9 increased as shown in Fig. 10. When the heating power increased, the reading of TC9 increased as well. From the experiment, with gas of argon as working fluid prototype B could dissipate 4W heat while the cold end temperature at the measurement point was below 6℃. The heating power in this experiment was much larger than those reported by other references[12,13], indicating the prototypes with current configurations could dissipate larger heat from electronic devices. Moreover, for most electronic devices, as their outside surface temperature are allowed much higher than several Celsius degree, more amount of heat can be dissipated with prototype B.Fig. 10 Reading of thermocouple no.9 varies with heating power5. CONCLUSIONSExperiments with two types of J-T micro-cooler were carried out to investigate their capability of electronic cooling. Both prototypes were fabricated with 7 layers (including the top and bottom layer) of microchannels. Gas of CO2 and argon were used as working fluid. Experiment results showed that for either CO2 or argon the higher the inlet gas pressure, the larger the temperature drop. And the lower the gas inlet temperature, the larger the temperature drop. The configuration of the prototype can be optimized to further increase the capability of heat removal. Hence, with further study gas throttling in microchannels with multilayer can be considered as a prospective method for cooling electronic devices with high-heat-flux.ACKNOWLEDGMENTThis research work was supported by Shanghai Natural Science Foundation, Grant No. 14ZR1429100. NOMENCLATUREKn Knudsen number ( - )L characteristic length (m)λmean free path (m)μJT Joule-Thompson coefficient (K/kPa)REFERENCES[1] Ebadian, M. A., Lin, C. X., “A review of high-heat-flux heat removal technologies,” J. of Heat Transfer, 133, pp.110801(1-11),(2011).[2] Ng, K. C., Xue, H., Wang, J.B., “Experimental and numerical study on a miniature Joule-Thomson cooler for steady-statecharacteristics, ”Int. J. Heat Mass Transf., 45, pp.609-618, (2002).[3] Maytal, B. Z., Nellis, G. F., Klein, S. A., et al., “Elevated-pressure mixed-coolants Joule-Thomson cryocooling,”Cryogenics,46, pp.55-67, (2006).[4] Timmerhaus, K. D., “ Cryocooler Development, ”AICHE Journal, 42(11), pp. 3203-3211, (1996).[5] Gong, M. Q., Wu, J. F., Yan, B., et al., “Study on a miniature mix-gases Joule-Thomson cooler driven by an oil-lubricatedmini-compressor for 120K temperature ranges,” Physics Procedia, 67, pp.405-410, (2015).[6] Derking, J. H., Vermeer, C. H., Tirolien, T., et al., “A mixed gas miniature Joule-Thomson cooling system,”Cryogenics, 57,pp.26-30, (2013).[7] Ardhapurkar, P. M., Sridharan, A., Atrey, M. D., “Experimental investigation on temperature profile and pressure drop in two-phase heat exchanger for mixed refrigerant Joule-Thomson cryocooler,”Applied Thermal Engineering, 66, pp.94-103, (2014).[8] Naer, V., Rozhentsev, A., “Application of hydrocarbon mixtures in small refrigerating and cryogenic machines, ”Int. J. ofRefigeration, 25, pp. 836-847, (2002).[9] Tuckerman, D. B., Pease, R. F. W. , “High performance heat sink for VLSI,” IEEE Electron Dev. Lett., EDL-2, pp. 126-129,(1981).[10] Colgan, E. G., Furman, B., Gaynes, M., et al., “A practical implementation of silicon microchannel coolers for high powerchips,”21st IEEE SEMI-THERM Symposium, pp. 1-7, (2005).[11] Wei, X., Joshi, Y., Patterson, M. K., “Experimental and numerical study of a stacked microchannel heat sink for liquid coolingof microelectronic devices, ”J. of Heat Transfer, 129, pp.1432-1444, (2007).[12] Little, W. A., “Microminiature refrigeration,”Advances in Cryogenic Engineering: Transactions of the Cryogenic EngineeringConference, 53: pp.597-605, (2008).[13] Lerou, P.P.P.M., Venhorst, G.C.F., Veenstra, T.T., et al., “All-micromachined Joule-Thomson cold stage,”Crycocoolers, 14,pp.437-441, (2007).[14] Hatwar, R., Ambad, S., Kumbhalkar, M. D., et al., “Development of a miniature Joule-Thomson refrigerator using LTCC(LowTemperature Co-fired Ceramic) technique,”Proceedings of 1st Int. Sym. on Physics and Technology of Sensors, pp.145-148, (2012).[15] Wang, Y. D., Lewis, R., Lin, M., et al., “Wafer-level processing for polymer-based planar micro cryogenic coolers,”25th IEEEInt. Conf. on Micro Electro Mechanical Systems, pp.341-344, (2012).[16] Kandlikar, S.,Garimella, S., Li,D., et al., Heat Transfer and Fluid Flow in Minichannels and Microchannels, Oxford: ElsevierInc., (2006).[17] Cengel, Y. A., Boles, M. A., Thermodynamics: An Engineering Approach, Fourth Ed.,New York: McGraw-Hill, (2002).。