Recent advances in conjugated polymer energy storage
共轭聚合物 有机半导体 英文
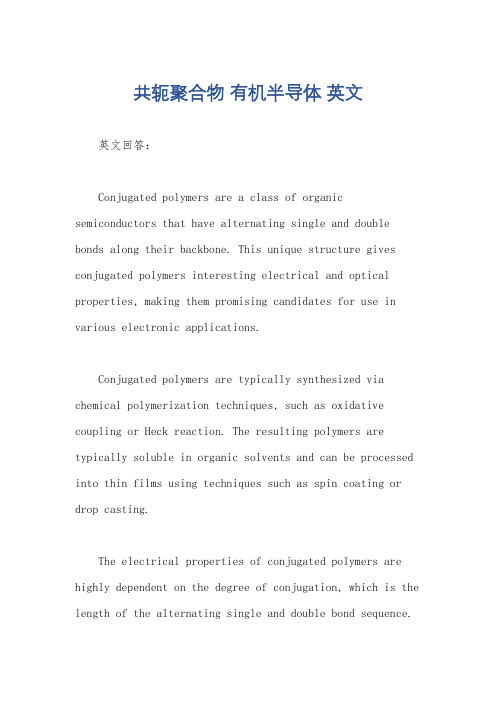
共轭聚合物有机半导体英文英文回答:Conjugated polymers are a class of organic semiconductors that have alternating single and double bonds along their backbone. This unique structure gives conjugated polymers interesting electrical and optical properties, making them promising candidates for use in various electronic applications.Conjugated polymers are typically synthesized via chemical polymerization techniques, such as oxidative coupling or Heck reaction. The resulting polymers are typically soluble in organic solvents and can be processed into thin films using techniques such as spin coating or drop casting.The electrical properties of conjugated polymers are highly dependent on the degree of conjugation, which is the length of the alternating single and double bond sequence.Longer conjugation lengths lead to higher charge carrier mobility and lower bandgap, making the polymer more conductive and semiconducting, respectively.The optical properties of conjugated polymers are also affected by the degree of conjugation. Longer conjugation lengths lead to absorption and emission of light at longer wavelengths, resulting in a red shift in the polymer's absorption and emission spectra.Conjugated polymers have been used in a variety of electronic applications, including organic solar cells, organic light-emitting diodes (OLEDs), and transistors. In organic solar cells, conjugated polymers act as the active layer, absorbing light and generating charge carriers that are then collected by the electrodes. In OLEDs, conjugated polymers are used as the emitting layer, emitting light when an electric current is applied. In transistors, conjugated polymers are used as the semiconductor channel, controlling the flow of current between the source and drain electrodes.Conjugated polymers are a promising class of materials for use in electronic applications due to their unique electrical and optical properties. Further research is needed to improve the performance and stability of conjugated polymers, but they have the potential to revolutionize the field of electronics.中文回答:共轭聚合物是有机半导体的一种,其主链上交替排列着单键和双键。
浙江大学化学系求是学者黄建国教授诚聘博士后研究人员
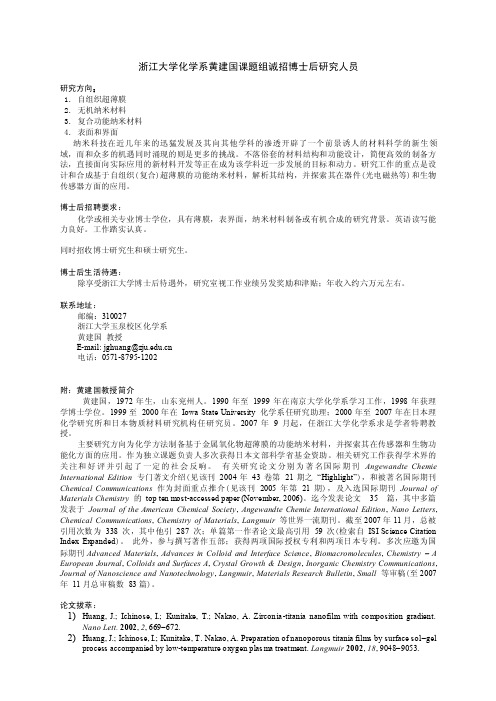
浙江大学化学系黄建国课题组诚招博士后研究人员研究方向:1. 自组织超薄膜2. 无机纳米材料3. 复合功能纳米材料4. 表面和界面纳米科技在近几年来的迅猛发展及其向其他学科的渗透开辟了一个前景诱人的材料科学的新生领域,而和众多的机遇同时涌现的则是更多的挑战。
不落俗套的材料结构和功能设计,简便高效的制备方法,直接面向实际应用的新材料开发等正在成为该学科近一步发展的目标和动力。
研究工作的重点是设计和合成基于自组织(复合)超薄膜的功能纳米材料,解析其结构,并探索其在器件(光电磁热等)和生物传感器方面的应用。
博士后招聘要求:化学或相关专业博士学位,具有薄膜,表界面,纳米材料制备或有机合成的研究背景。
英语读写能力良好。
工作踏实认真。
同时招收博士研究生和硕士研究生。
博士后生活待遇:除享受浙江大学博士后待遇外,研究室视工作业绩另发奖励和津贴;年收入约六万元左右。
联系地址:邮编:310027浙江大学玉泉校区化学系黄建国教授E-mail:***************.cn电话:0571-8795-1202附:黄建国教授简介黄建国,1972 年生,山东兖州人。
1990 年至1999 年在南京大学化学系学习工作,1998 年获理学博士学位。
1999 至2000 年在Iowa State University化学系任研究助理;2000 年至2007 年在日本理化学研究所和日本物质材料研究机构任研究员。
2007 年9 月起,任浙江大学化学系求是学者特聘教授。
主要研究方向为化学方法制备基于金属氧化物超薄膜的功能纳米材料,并探索其在传感器和生物功能化方面的应用。
作为独立课题负责人多次获得日本文部科学省基金资助。
相关研究工作获得学术界的关注和好评并引起了一定的社会反响。
有关研究论文分别为著名国际期刊Angewandte Chemie International Edition专门著文介绍(见该刊2004年43卷第21期之“Highlight”),和被著名国际期刊Chemical Communications作为封面重点推介(见该刊2005年第21 期),及入选国际期刊Journal of Materials Chemistry的top ten most-accessed paper (November, 2006)。
氟尿嘧啶大分子前体药物肿瘤靶向的研究进展
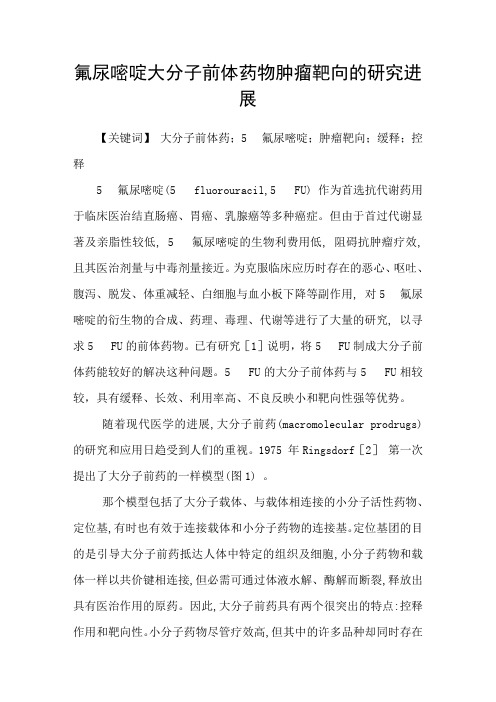
氟尿嘧啶大分子前体药物肿瘤靶向的研究进展【关键词】大分子前体药;5氟尿嘧啶;肿瘤靶向;缓释;控释5氟尿嘧啶(5fluorouracil,5FU) 作为首选抗代谢药用于临床医治结直肠癌、胃癌、乳腺癌等多种癌症。
但由于首过代谢显著及亲脂性较低, 5氟尿嘧啶的生物利费用低, 阻碍抗肿瘤疗效, 且其医治剂量与中毒剂量接近。
为克服临床应历时存在的恶心、呕吐、腹泻、脱发、体重减轻、白细胞与血小板下降等副作用, 对5氟尿嘧啶的衍生物的合成、药理、毒理、代谢等进行了大量的研究, 以寻求5FU的前体药物。
已有研究[1]说明,将5FU制成大分子前体药能较好的解决这种问题。
5FU的大分子前体药与5FU相较较,具有缓释、长效、利用率高、不良反映小和靶向性强等优势。
随着现代医学的进展,大分子前药(macromolecular prodrugs) 的研究和应用日趋受到人们的重视。
1975 年Ringsdorf[2]第一次提出了大分子前药的一样模型(图1) 。
那个模型包括了大分子载体、与载体相连接的小分子活性药物、定位基,有时也有效于连接载体和小分子药物的连接基。
定位基团的目的是引导大分子前药抵达人体中特定的组织及细胞,小分子药物和载体一样以共价键相连接,但必需可通过体液水解、酶解而断裂,释放出具有医治作用的原药。
因此,大分子前药具有两个很突出的特点:控释作用和靶向性。
小分子药物尽管疗效高,但其中的许多品种却同时存在着专门大的副作用。
在它们进入人体后的短时刻内,血液中药物的浓度远远超过医治所需的浓度,太高的浓度可能引发很多副作用,同时它们在生物体内新陈代谢速度快,半衰期短,易排泄,故随着时刻的推延,药物的浓度降低较快,阻碍疗效;另一方面,小分子药物对进入体内指定部位也缺乏选择性,这也是给药量增多、疗效较低、副作用大的缘故之一[3]。
基于这些缘故,最近几年来药物释放体系(DDS) 已成为热点研究领域,大分子前药在其中占据了很重要的位置。
全激基复合物发光实现结构简单、光谱稳定白色有机电致发光器件

Fig. 1 The structure of devices A—D and the chemical structures of mCPꎬTAPC and PO ̄T2T
1 实验
在相 同 实 验 条 件 下ꎬ 制 备 的 器 件 结 构 为 ITO / HAT ̄CN (10 nm) / TAPC (50 nm) / mCP (10 nm) /
图 4 ( A) 器件 R2 的归一化电致发光光谱( 电流密度为 20 mA / cm2 ) ꎬ插图为器件 R2 的结构ꎻ( B) TAPC 和 PO ̄T2T
的能级结构及形成激基复合物示意图
Fig. 4 ( A) Normalized EL spectra of devices R2 at 20 mA / cm2 and inset shows the structure of device R2ꎬ( B) Scheme
0 引言
白色有机发光二极管( White Organic Light ̄Emitting DiodesꎬWOLEDs) 在柔性、大面积照明领域的应
用中受到了特别的关注 [1 ̄2] . 全磷光 WOLEDs 由于含有重金属配合物的磷光分子具有 100% 的激子利用
率ꎬ通过合理的器件结构设计ꎬ其器件效率可以接近理论极限值 [3 ̄4] . 然而ꎬ磷光染料高昂的价格及相对
吻合. 这表明此两种混合染料之间同样形成了发射橙光的激基复合物. 这些结果表明图 2 器件 A—D 所
第3 期
董 贺ꎬ等:全激基复合物发光实现结构简单、光谱稳定白色有机电致发光器件
75
获得的蓝色和橙色发光峰为激基复合物发射ꎬ另外橙光光谱的蓝移主要与分子间作用有关 [12] .
国外碳纳米管复合材料研究现状
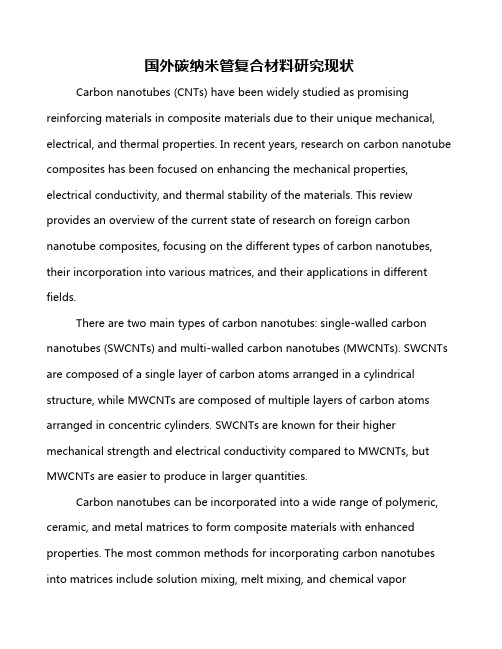
国外碳纳米管复合材料研究现状Carbon nanotubes (CNTs) have been widely studied as promising reinforcing materials in composite materials due to their unique mechanical, electrical, and thermal properties. In recent years, research on carbon nanotube composites has been focused on enhancing the mechanical properties, electrical conductivity, and thermal stability of the materials. This review provides an overview of the current state of research on foreign carbon nanotube composites, focusing on the different types of carbon nanotubes, their incorporation into various matrices, and their applications in different fields.There are two main types of carbon nanotubes: single-walled carbon nanotubes (SWCNTs) and multi-walled carbon nanotubes (MWCNTs). SWCNTs are composed of a single layer of carbon atoms arranged in a cylindrical structure, while MWCNTs are composed of multiple layers of carbon atoms arranged in concentric cylinders. SWCNTs are known for their higher mechanical strength and electrical conductivity compared to MWCNTs, but MWCNTs are easier to produce in larger quantities.Carbon nanotubes can be incorporated into a wide range of polymeric, ceramic, and metal matrices to form composite materials with enhanced properties. The most common methods for incorporating carbon nanotubes into matrices include solution mixing, melt mixing, and chemical vapordeposition. The choice of matrix material and processing method can greatly affect the properties of the resulting composite material.One of the key advantages of carbon nanotube composites is their exceptional mechanical properties. The high aspect ratio and strong covalent bonds of carbon nanotubes make them ideal reinforcing materials for improving the strength and stiffness of composite materials. Studies have shown that the addition of carbon nanotubes can significantly increase the tensile strength, flexural strength, and impact resistance of composite materials. Additionally, carbon nanotubes can also improve the toughness and fatigue resistance of composite materials, making them suitable for a wide range of structural applications.In addition to their mechanical properties, carbon nanotube composites are also known for their excellent electrical conductivity. Carbon nanotubes have a high electrical conducti vity due to their delocalized π-electron system, making them ideal materials for applications in electronics, sensors, and energy storage devices. Studies have shown that the addition of carbon nanotubes can significantly increase the electrical conductivity of composite materials, making them suitable for use in conductive coatings, electromagnetic shielding, and antistatic materials.Furthermore, carbon nanotube composites also exhibit enhanced thermal stability due to the excellent thermal conductivity of carbon nanotubes. Thehigh thermal conductivity of carbon nanotubes allows for efficient heat transfer within the composite material, leading to improved thermal management and heat dissipation properties. Studies have shown that the addition of carbon nanotubes can significantly increase the thermal conductivity and heat resistance of composite materials, making them suitable for applications in aerospace, automotive, and electronic devices.Overall, research on foreign carbon nanotube composites has shown promising results in enhancing the mechanical, electrical, and thermal properties of composite materials. The unique properties of carbon nanotubes make them ideal reinforcing materials for improving the performance of composite materials in various applications. Further research is needed to optimize the processing methods and matrix materials to fully exploit the potential of carbon nanotube composites in different fields.。
Conjugated Polymer Dots-on-Electrospun Fibers as a Fluorescent
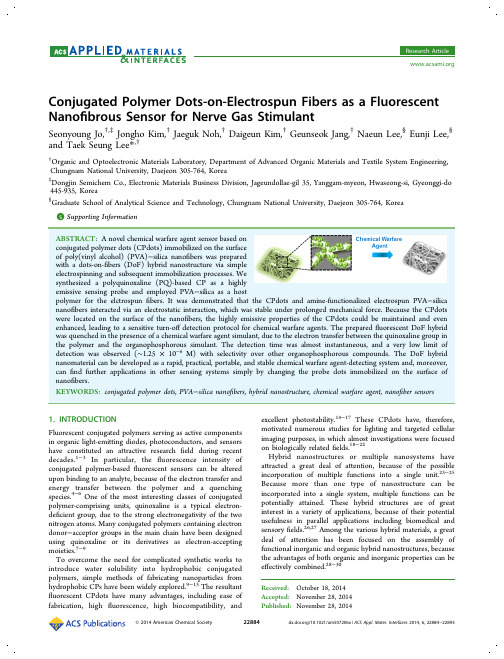
1. INTRODUCTION Fluorescent conjugated polymers serving as active components in organic light-emitting diodes, photoconductors, and sensors have constituted an attractive research field during recent decades.1−3 In particular, the fluorescence intensity of conjugated polymer-based fluorescent sensors can be altered upon binding to an analyte, because of the electron transfer and energy transfer between the polymer and a quenching species.4−6 One of the most interesting classes of conjugated polymer-comprising units, quinoxaline is a typical electrondeficient group, due to the strong electronegativity of the two nitrogen atoms. Many conjugated polymers containing electron donor−acceptor groups in the main chain have been designed using quinoxaline or its derivatives as electron-accepting moieties.7−9 To overcome the need for complicated synthetic works to introduce water solubility into hydrophobic conjugated polymers, simple methods of fabricating nanoparticles from hydrophobic CPs have been widely explored.9−13 The resultant fluorescent CPdots have many advantages, including ease of fabrication, high fluorescence, high biocompatibility, and
Gaussian09在材料科学中的应用
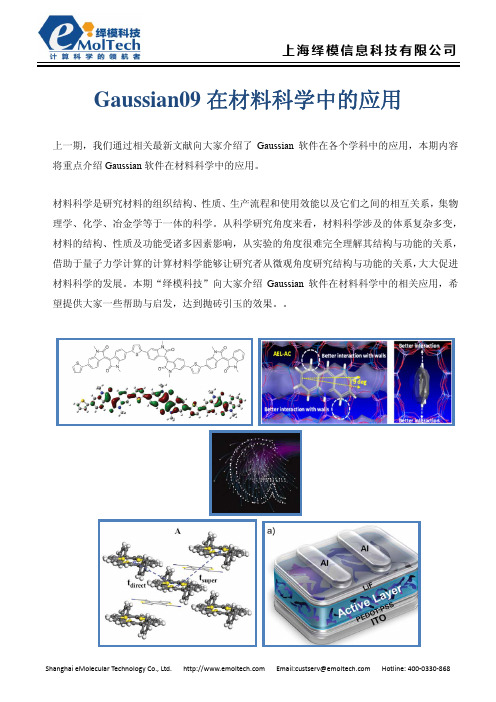
Grancini, G. et. al. Hot exciton dissociation in polymer solar cells. Nature materials. 2013, 12 (1), 29-33. Shanghai eMolecular Technology Co., Ltd. Email:custserv@ Hotline: 400‐0330‐868
Shanghai eMolecular Technology Co., Ltd. Email:custserv@ Hotline: 400‐0330‐868
上海绎模信息科技有限公司
Gaussian 功能列表
- 研究化学反应
• 平衡结构及过渡态结构确定 • 势能面分析 • 基元反应能垒 • 反应热 • 反应机理
Shanghai eMolecular Technology Co., Ltd. Email:custserv@ Hotline: 400‐0330‐868
上海绎模信息科技有限公司
应用范例 5:
本期内容
• 在所有的有机光伏太阳能电池系统中,光诱导动力学是光电转换供受界面上最重要的过程。 然而到目前为止,对于光电转换这一过程中界面电荷分离的细致机理,研究者还并未达成 共识。本文作者发现在 PCPDTBT/PC60BM 异质结体系中,单重态可在 20~50fs 内转化为 电荷转移态及极化子。
Shanghai eMolecular Technology Co., Ltd. Email:custserv@ Hotline: 400‐0330‐868
基于宽带隙聚合物太阳能电池的研究进展

第49卷第9期2021年5月广州化工Guangzhou Chemical IndustryVol. 49 No. 9May. 2021基于宽带隙聚合物太阳能电池的研究进展庄程东郭鹏智2(1兰州交通大学材料科学与工程学院,甘肃 兰州730070;2兰州交通大学国家绿色镀膜技术与装备工程技术研究中心,甘肃 兰州730070)摘 要:有机太阳能电池因为制备过程简单、重量轻、成本低廉和可制成柔性器件等优点受到了广泛的关注。
近年来,随着窄带隙小分子受体材料的快速发展,与之光谱匹配的宽带隙给体聚合物太阳能电池表现出较高的PCE 。
研究人员通过苯并二廛 吩(BDT)、苯并二嗟吩-4,8-二酮(BDD)、蔡并双三醴和苯并三®(BTA)等构建块,设计合成了一系列高效的宽带隙给体聚合物。
在此对宽带隙聚合物非富勒烯太阳能电池的研究进展进行综述。
关键词:给体聚合物;有机太阳能电池;宽带隙;研究进展中图分类号:0631.3文献标志码:A 文章编号: 1001-9677 (2021) 09-0021-07Research Progress on Wide Bandgap Polymers for Polymer Solar Cells **基金项目:国家自然科学基金(No. 51903112)。
第一作者:庄程东,男,硕士,研究方向:有机太阳能电池材料的合成与研究。
通讯作者:郭鹏智,男,博士,研究方向:有机太阳能电池材料的设计、合成与器件研究。
ZHUANG Cheng-dong 1, GUO Peng-zhi'(1 School of Materials Scienceand Engineering , Lanzhou Jiaotong University , Gansu Lanzhou 730070 ;2 National Green Coating Technology and Equipment Research Center , Lanzhou Jiaotong University ,Gansu Lanzhou 730070, China)Abstract : Organic solar cells have attracted much attention because of their excellent advantages of easy fabrication ,light-weight , low-cost and flexibility. In recent years , with the rapid development of narrow-band gap small molecularacceptor materials , the wide band gap donor polymer solar cells with spectrum matching show higher PCE. A series ofhigh efficient wide band gap donor polymers were designed and synthesized by using benzodithiophene ( BDT ), benzodithiophene -4,8 - dione ( BDD) , naphthalene bistriazole ( TZNT ) and benztriazole ( BTA ) as building blocks. Theresearch progress onnon fullerene organic solar cells with wide gap donor polymers was reviewed.Key words : donorpolymers ; organic solar cell ; wide band gap ; progress有机太阳能电池因为其成本低、重量轻、柔性、可大面积印刷制备等优点成为研究热点。
共轭聚合物 有机半导体 英文

共轭聚合物有机半导体英文英文回答:Conjugated polymers and organic semiconductors are a class of materials that have both semiconducting and polymeric properties. They are typically composed of alternating single and double bonds along the polymer backbone, which allows for the delocalization of electrons and the formation of pi-conjugated systems. This delocalization results in a number of unique properties, including high electrical conductivity, low optical bandgaps, and the ability to absorb and emit light.Conjugated polymers and organic semiconductors have a wide range of potential applications in electronic devices, such as solar cells, light-emitting diodes (LEDs), and transistors. They are also being investigated for use in sensors, batteries, and other energy-related applications.中文回答:共轭聚合物和有机半导体是一类既具有半导体特性又具有聚合物性质的材料。
Polymer Science Advances and Applications
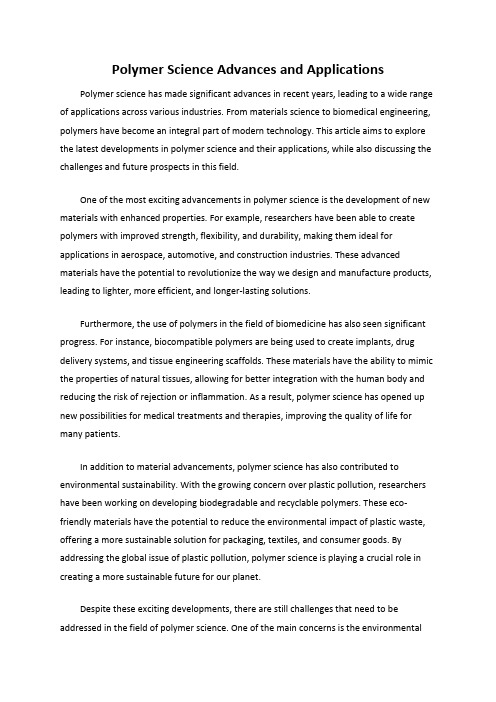
Polymer Science Advances and Applications Polymer science has made significant advances in recent years, leading to a wide range of applications across various industries. From materials science to biomedical engineering, polymers have become an integral part of modern technology. This article aims to explore the latest developments in polymer science and their applications, while also discussing the challenges and future prospects in this field.One of the most exciting advancements in polymer science is the development of new materials with enhanced properties. For example, researchers have been able to create polymers with improved strength, flexibility, and durability, making them ideal for applications in aerospace, automotive, and construction industries. These advanced materials have the potential to revolutionize the way we design and manufacture products, leading to lighter, more efficient, and longer-lasting solutions.Furthermore, the use of polymers in the field of biomedicine has also seen significant progress. For instance, biocompatible polymers are being used to create implants, drug delivery systems, and tissue engineering scaffolds. These materials have the ability to mimic the properties of natural tissues, allowing for better integration with the human body and reducing the risk of rejection or inflammation. As a result, polymer science has opened up new possibilities for medical treatments and therapies, improving the quality of life for many patients.In addition to material advancements, polymer science has also contributed to environmental sustainability. With the growing concern over plastic pollution, researchers have been working on developing biodegradable and recyclable polymers. These eco-friendly materials have the potential to reduce the environmental impact of plastic waste, offering a more sustainable solution for packaging, textiles, and consumer goods. By addressing the global issue of plastic pollution, polymer science is playing a crucial role in creating a more sustainable future for our planet.Despite these exciting developments, there are still challenges that need to be addressed in the field of polymer science. One of the main concerns is the environmentalimpact of polymer production and disposal. While biodegradable polymers offer a promising solution, their large-scale production and commercialization still pose technical and economic challenges. Additionally, the recycling of polymers remains a complex and costly process, hindering widespread adoption and implementation.Looking ahead, the future of polymer science holds great potential for further innovation and discovery. Researchers are constantly exploring new polymerization techniques, advanced characterization methods, and novel applications. With the rapid advancement of technology, such as 3D printing and nanotechnology, the possibilities for creating new and improved polymer materials are endless. Furthermore, the interdisciplinary nature of polymer science, combining chemistry, physics, engineering, and biology, opens up opportunities for collaboration and cross-disciplinary research, leading to even more groundbreaking advancements.In conclusion, polymer science has made significant strides in recent years, leading to a wide range of applications and advancements across various industries. From materials with enhanced properties to biocompatible and eco-friendly solutions, polymers have the potential to revolutionize the way we live, work, and interact with our environment. While there are still challenges to be addressed, the future of polymer science looks promising, with endless opportunities for further innovation and discovery. As researchers continue to push the boundaries of what is possible, the impact of polymer science on our daily lives is expected to grow exponentially, shaping a more sustainable and technologically advanced future.。
国外碳纳米管复合材料研究现状
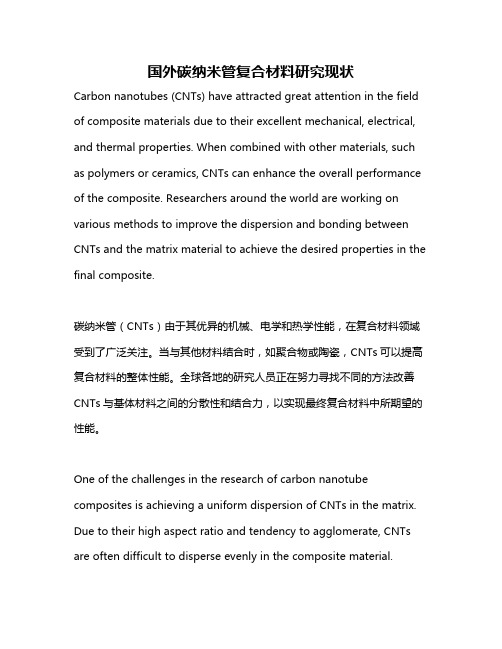
国外碳纳米管复合材料研究现状Carbon nanotubes (CNTs) have attracted great attention in the field of composite materials due to their excellent mechanical, electrical, and thermal properties. When combined with other materials, such as polymers or ceramics, CNTs can enhance the overall performance of the composite. Researchers around the world are working on various methods to improve the dispersion and bonding between CNTs and the matrix material to achieve the desired properties in the final composite.碳纳米管(CNTs)由于其优异的机械、电学和热学性能,在复合材料领域受到了广泛关注。
当与其他材料结合时,如聚合物或陶瓷,CNTs可以提高复合材料的整体性能。
全球各地的研究人员正在努力寻找不同的方法改善CNTs与基体材料之间的分散性和结合力,以实现最终复合材料中所期望的性能。
One of the challenges in the research of carbon nanotube composites is achieving a uniform dispersion of CNTs in the matrix. Due to their high aspect ratio and tendency to agglomerate, CNTs are often difficult to disperse evenly in the composite material.Improper dispersion can lead to weak interfacial bonding, which limits the enhancement of mechanical properties. Various techniques, such as functionalization of CNTs or the use of surfactants, have been explored to improve dispersion and enhance the overall performance of the composite.碳纳米管复合材料研究中的一个挑战是实现CNTs在基体中的均匀分散。
The properties of conjugated polymers
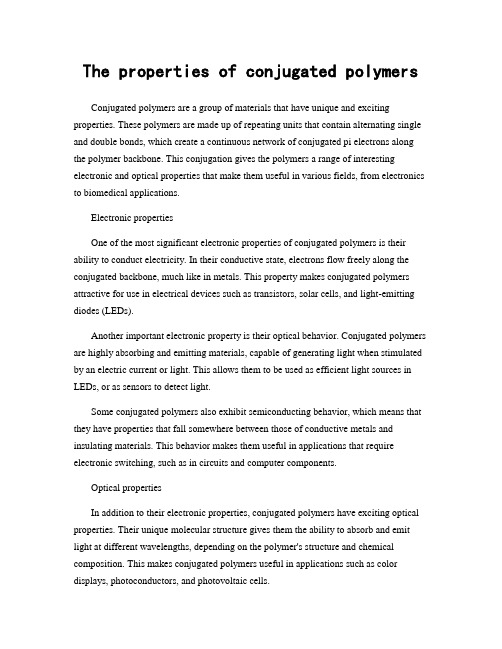
The properties of conjugated polymersConjugated polymers are a group of materials that have unique and exciting properties. These polymers are made up of repeating units that contain alternating single and double bonds, which create a continuous network of conjugated pi electrons along the polymer backbone. This conjugation gives the polymers a range of interesting electronic and optical properties that make them useful in various fields, from electronics to biomedical applications.Electronic propertiesOne of the most significant electronic properties of conjugated polymers is their ability to conduct electricity. In their conductive state, electrons flow freely along the conjugated backbone, much like in metals. This property makes conjugated polymers attractive for use in electrical devices such as transistors, solar cells, and light-emitting diodes (LEDs).Another important electronic property is their optical behavior. Conjugated polymers are highly absorbing and emitting materials, capable of generating light when stimulated by an electric current or light. This allows them to be used as efficient light sources in LEDs, or as sensors to detect light.Some conjugated polymers also exhibit semiconducting behavior, which means that they have properties that fall somewhere between those of conductive metals and insulating materials. This behavior makes them useful in applications that require electronic switching, such as in circuits and computer components.Optical propertiesIn addition to their electronic properties, conjugated polymers have exciting optical properties. Their unique molecular structure gives them the ability to absorb and emit light at different wavelengths, depending on the polymer's structure and chemical composition. This makes conjugated polymers useful in applications such as color displays, photoconductors, and photovoltaic cells.Moreover, conjugated polymers have a high degree of photostability, which means that they maintain their optical properties even after prolonged exposure to light. This property makes them ideal for use in long-lasting products such as outdoor signs and displays.Mechanical propertiesIn addition to their electronic and optical properties, conjugated polymers have excellent mechanical properties. Their inherent polymer chains make them flexible, sturdy, and resistant to wear and tear. This property makes them attractive for use in devices that require flexibility, such as in electronic circuits or biomedical applications.Moreover, conjugated polymers can be synthesized with properties that allow them to exhibit shape-memory behavior, which is the ability to return to their original shape after being deformed. This property facilitates the development of deformable electronic devices such as flexible displays, stretchable sensors, and wearable electronics.Biomedical propertiesApart from electronics and optics, conjugated polymers also have promising properties in biomedical applications. Their ability to interact with biological systems makes them a potential candidate for use in drug delivery, bioimaging, and tissue engineering.One of the most significant biomedical properties of conjugated polymers is their ability to interact with ions and molecules in biological systems. This property makes them useful in detecting and analyzing biomolecules such as proteins, nucleic acids, and enzymes.Another crucial property is conjugated polymers' biocompatibility, which allows them to coexist with living organisms without causing any adverse effects. This property makes them a potential material for use in implants and other medical devices.ConclusionIn summary, conjugated polymers are a unique class of materials that exhibit a range of fascinating properties, including electronic, optical, and mechanical properties. Their versatility has led to their use in a wide range of applications, from electronics to biomedical applications, and their potential is still being explored in various areas. With ongoing research, we can expect to see even more exciting developments that harness the power of conjugated polymers and their properties.。
CONJUGATEDPOLYMERSWITHHIGHLYPOLARSIDECHAIN..
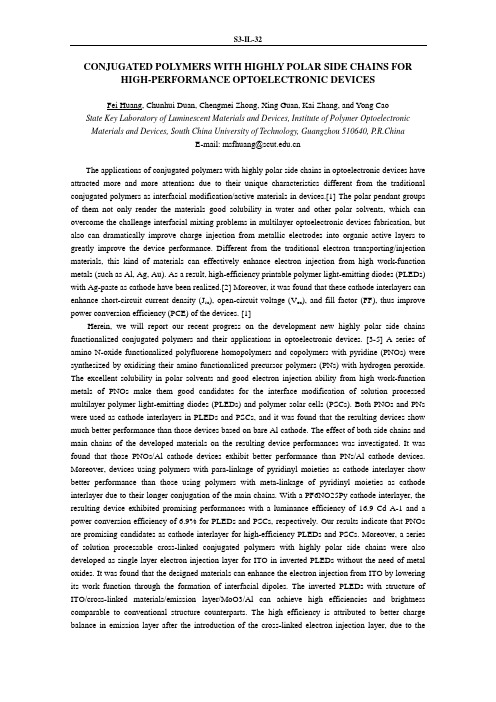
CONJUGATED POLYMERS WITH HIGHLY POLAR SIDE CHAINS FOR HIGH-PERFORMANCE OPTOELECTRONIC DEVICESFei Huang, Chunhui Duan, Chengmei Zhong, Xing Guan, Kai Zhang, and Yong Cao State Key Laboratory of Luminescent Materials and Devices, Institute of Polymer OptoelectronicMaterials and Devices, South China University of Technology, Guangzhou 510640, P.R.ChinaE-mail:*****************.cnThe applications of conjugated polymers with highly polar side chains in optoelectronic devices have attracted more and more attentions due to their unique characteristics different from the traditional conjugated polymers as interfacial modification/active materials in devices.[1] The polar pendant groups of them not only render the materials good solubility in water and other polar solvents, which can overcome the challenge interfacial mixing problems in multilayer optoelectronic devices fabrication, but also can dramatically improve charge injection from metallic electrodes into organic active layers to greatly improve the device performance. Different from the traditional electron transporting/injection materials, this kind of materials can effectively enhance electron injection from high work-function metals (such as Al, Ag, Au). As a result, high-efficiency printable polymer light-emitting diodes (PLEDs) with Ag-paste as cathode have been realized.[2] Moreover, it was found that these cathode interlayers can enhance short-circuit current density (J sc), open-circuit voltage (V oc), and fill factor (FF), thus improve power conversion efficiency (PCE) of the devices. [1]Herein, we will report our recent progress on the development new highly polar side chains functionalized conjugated polymers and their applications in optoelectronic devices. [3-5] A series of amino N-oxide functionalized polyfluorene homopolymers and copolymers with pyridine (PNOs) were synthesized by oxidizing their amino functionalized precursor polymers (PNs) with hydrogen peroxide. The excellent solubility in polar solvents and good electron injection ability from high work-function metals of PNOs make them good candidates for the interface modification of solution processed multilayer polymer light-emitting diodes (PLEDs) and polymer solar cells (PSCs). Both PNOs and PNs were used as cathode interlayers in PLEDs and PSCs, and it was found that the resulting devices show much better performance than those devices based on bare Al cathode. The effect of both side chains and main chains of the developed materials on the resulting device performances was investigated. It was found that those PNOs/Al cathode devices exhibit better performance than PNs/Al cathode devices. Moreover, devices using polymers with para-linkage of pyridinyl moieties as cathode interlayer show better performance than those using polymers with meta-linkage of pyridinyl moieties as cathode interlayer due to their longer conjugation of the main chains. With a PF6NO25Py cathode interlayer, the resulting device exhibited promising performances with a luminance efficiency of 16.9 Cd A-1 and a power conversion efficiency of 6.9% for PLEDs and PSCs, respectively. Our results indicate that PNOs are promising candidates as cathode interlayer for high-efficiency PLEDs and PSCs. Moreover, a series of solution processable cross-linked conjugated polymers with highly polar side chains were also developed as single layer electron injection layer for ITO in inverted PLEDs without the need of metal oxides. It was found that the designed materials can enhance the electron injection from ITO by lowering its work function through the formation of interfacial dipoles. The inverted PLEDs with structure of ITO/cross-linked materials/emission layer/MoO3/Al can achieve high efficiencies and brightness comparable to conventional structure counterparts. The high efficiency is attributed to better charge balance in emission layer after the introduction of the cross-linked electron injection layer, due to theelectron injection enhancement brought by amino alkyl pendant group containing side chains and the hole blocking, leakage current suppressing ability brought by the polyfluorene main chain. These findings provide a new way to design high performance solution processed inverted optoelectronic devices.AcknowledgementsThis work was supported by Ministry of Science and Technology Project (No.2009CB623600) and the Natural Science Foundation of China (Project No. 21125419, 50990065).References(1)Huang, F.; Wu, H.B.; Cao, Y. Chem. Soc. Rev.2010, 39, 2500(2)Zeng, W. J.; Wu, H. B.; Zhang, C.; Huang, F.; Peng, J.B.; Yang, W.; Cao, Y. Adv. Mater. 2007, 19,810.(3)Duan, C. H.; Wang, L.; Zhang, K.; Guan, X.; Huang F. Adv. Mater.2011, 23, 1665.(4)Zhong, C. M.; Liu, S. J.; Huang, F.; Wu, H. B.; Cao, Y. Chem. Mater., 2011, 23, 4870.(5)Guan, X.; Zhang, K.; Huang, F.; Bazan, G. C.;Cao, Y. Adv. Funct. Mater., 2012,10.1002/adfm.201200199.。
美国FDA细菌学分析手册第八版(BAM)食源性病原体快速检测方法

BAM: Rapid Methods for Detecting Foodborne PathogensJanuary 2001Bacteriological Analytical ManualAppendix 1Rapid Methods for Detecting Foodborne PathogensAuthorsIntroductionAuthors Note: This section differs from others in this manual in that it lists methods that are not necessarily used by FDA. In addition, the detailed protocols for these methods are not presented, and the user is referred to the instructions that accompany the test kits. One reason for this departure is the incremental rate of change and innovation in rapid testing technology. The best of these new techniques should be evaluated individually by user labs for their particular needs, and also collaboratively for possible adoption as official methods by the AOAC International (1).The following text and tables list many of the commercially available rapid methods; they are classified by the principles underlying the procedure used. The assay principles and some of the detailed procedures are discussed in other chapters of this manual and/or in the literature cited in the tables. The AOAC status of rapid tests is indicated for those methods that have been validated or evaluated by AOAC (1) and have been adopted as AOAC Official methods. However, these methods continue to be modified or adapted, so that published information may not be the most current. Rapid methods are generally used as screening techniques, with negative results accepted as is, but positive results requiring confirmation by the appropriate official method, which, in many instances, is cultural. In many other instances, the rapid method has not been validated; therefore, the listing of a method or kit in this chapter in no way constitutes FDA recommendation or approval.Rapid MethodsThe rapid detection of pathogens and other microbial contaminants in food is critical for ensuring the safety of consumers. Traditional methods to detect foodborne bacteria often rely on time-consuming growth in culture media, followed by isolation, biochemical identification, and sometimes serology. Recent advances in technology make detection and identification faster, more convenient, more sensitive, and more specific than conventional assays -- at least in theory. These new methods are often referred to as "rapid methods", a subjective term used loosely to describe a vast array of tests that includes miniaturized biochemical kits, antibody- and DNA-based tests, and assays that are modifications of conventional tests to speed up analysis (8, 15, 16, 24, 36). Some of these assays have also been automated to reduce hands-on manipulations. With few exceptions, almost all assays used to detect specific pathogens in foods require some growth in an enrichment medium before analysis.Experts who were surveyed in 1981 (19) about future developments in methods used for food microbiology, accurately predicted the widespread use of miniaturized biochemical kits for the identification of pure cultures of bacteria isolated from food. Most consist of a disposable device containing 15 - 30 media or substrates specifically designed to identify a bacterial group or species. With the exception of a few kits where results can be read in 4 hrs, most require 18-24 hrs incubation. In general, miniaturized biochemical tests are very similar in format and performance, showing 90-99% accuracy in comparison to conventional methods (5, 16, 21). However, kits that have been in use longer may have a more extensive identification database than newer tests. Most miniaturized kits are designedfor enteric bacteria, but kits for the identification of non-Enterobacteriaceae are also available, including for Campylobacter, Listeria, anaerobes, non-fermenting Gram-negative bacteria and for Gram-positive bacteria (Table 1).Advances in instrumentation have enabled automation of the miniaturized biochemical identification tests. These instruments can incubate the reactions and automatically monitor biochemical changes to generate a phenotypic profile, which is then compared with the provided database stored in the computer to provide an identification (8, 23, 35). Other automated systems identify bacteria based on compositional or metabolic properties, such as fatty acid profiles, carbon oxidation profiles (28) or other traits (Table 1). Not forecast in that 1981 survey were the potential applications of immunological and genetic techniques in food microbiology (19). During the 1980s, major advances in basic research were transferred rapidly to applied areas, as "biotechnology" companies emerged and sought markets in the diagnostic field (11). DNA and antibody-based assays for numerous microbes or their toxins are now available commercially (12).There are many DNA-based assay formats, but only probes, PCR and bacteriophage have been developed commercially for detecting foodborne pathogens. Probe assays generally target ribosomal RNA (rRNA), taking advantage of the fact that the higher copy number of bacterial rRNA provides a naturally amplified target and affords greater assay sensitivity (6, 14, 25, 37) (Table 2).The basic principle of DNA hybridization is also being utilized in other technologies, such as the polymerase chain reaction (PCR) assay, where short fragments of DNA (probes) or primers are hybridized to a specific sequence or template, which is then enzymatically amplified by Taq polymerase using a thermocycler (2, 22). Theoretically, PCR can amplify a single copy of DNA by a million fold in less than 2 hrs; hence its potential to eliminate, or greatly reduce the need for cultural enrichment. However, the presence of inhibitors in foods and in many culture media can prevent primer binding and diminish amplification efficiency (26, 34), so that the extreme sensitivity achievable by PCR with pure cultures is often reduced when testing foods. Therefore, some cultural enrichment is still required prior to analysis (Table 2).The highly specific interaction of phage with its bacterial host has also been used to develop assays for foodborne pathogens (38). One example is an assay for Salmonella, in which a specific bacteriophage was engineered to carry a detectable marker (ice nucleation gene). In the presence of Salmonella, the phage confers the marker to the host, which then expresses the phenotype to allow detection (Table 2). The highly specific binding of antibody to antigen, especially monoclonal antibody, plus the simplicity and versatility of this reaction, has facilitated the design of a variety of antibody assays and formats, and they comprise the largest group of rapid methods being used in food testing (3, 10, 12, 33). There are 5 basic formats of antibody assays (12), the simplest of which is latex agglutination (LA), in which antibody-coated colored latex beads or colloidal gold particles are used for quick serological identification or typing of pure culture isolates of bacteria from foods (7, 12). A modification of LA, known as reverse passive latex agglutination (RPLA), tests for soluble antigens and is used mostly in testing for toxins in food extracts or for toxin production by pure cultures (12) (Table 3).In the immunodiffusion test format, an enrichment sample is placed in a gel matrix with the antibody; if the specific antigen is present, a visible line of precipitation is formed (30).The enzyme-linked immunosorbent assay (ELISA) is the most prevalent antibody assay format used for pathogen detection in foods (3, 33). Usually designed as a "sandwich" assay, an antibody bound to a solid matrix is used to capture the antigen from enrichment cultures and a second antibody conjugated to an enzyme is used for detection. The walls of wells in microtiter plates are the most commonly used solid support; but ELISAs have also been designed using dipsticks, paddles, membranes, pipet tips or other solid matrices (12) (Table 3).Antibodies coupled to magnetic particles or beads are also used in immunomagnetic separation (IMS) technology to capture pathogens from pre-enrichment media (31). IMS is analogous to selective enrichment, but instead of using antibiotics or harsh reagents that can cause stress-injury, an antibody is used to capture the antigen, which is a much milder alternative. Captured antigens can be plated or further tested using other assays.Immunoprecipitation or immunochromatography, still another antibody assay format, is based on the technology developed for home pregnancy tests. It is also a "sandwich" procedure but, instead of enzyme conjugates, the detection antibody is coupled to colored latex beads or to colloidal gold. Using only a 0.1 ml aliquot, the enrichment sample is wicked across a series of chambers to obtain results (9). These assays are extremely simple, require no washing or manipulation and are completed within 10 minutes after cultural enrichment (Table 3).The last mentioned "category" of rapid methods includes a large variety of assays, ranging from specialized media to simple modifications of conventional assays, which result in saving labor, time, and materials. Some, for instance, use disposable cardboards containing dehydrated media, which eliminates the need for agar plates, constituting savings in storage, incubation and disposal procedures (4, 5). Others incorporate specialized chromogenic and fluorogenic substrates in media to rapidly detect trait enzymatic activity (13, 17, 20, 27, 29). There are also tests that measure bacterial adenosine triphosphate (ATP), which (although not identifying specific species), can be used to rapidly enumerate the presence of total bacteria (Table 4).Applications and Limitations of Rapid MethodsAlmost all rapid methods are designed to detect a single target, which makes them ideal for use in quality control programs to quickly screen large numbers of food samples for the presence of a particular pathogen or toxin. A positive result by a rapid method however, is only regarded as presumptive and must be confirmed by standard methods (11). Although confirmation may extend analysis by several days, this may not be an imposing limitation, as negative results are most often encountered in food analysis.Most rapid methods can be done in a few minutes to a few hours, so they are more rapid than traditional methods. But, in food analysis, rapid methods still lack sufficient sensitivity and specificity for direct testing; hence, foods still need to be culture-enriched before analysis (12). Although enrichment is a limitation in terms of assay speed, it provides essential benefits, such as diluting the effects of inhibitors, allowing the differentiation of viable from non-viable cells and allowing for repair of cell stress or injury that may have resulted during food processing.Evaluations of rapid methods show that some perform better in some foods than others. This can be attributed mostly to interference by food components, some of which can be especially troublesome for the technologies used in rapid methods. For example, an ingredient can inhibit DNA hybridization or Taq polymerase, but has no effect on antigen-antibody interactions and the converse situation may also occur (12). Since method efficiencies may be food dependent, it is advisable to perform comparative studies to ensure that a particular assay will be effective in the analysis of that food type.The specificity of DNA based assays is dictated by short probes; hence, a positive result, for instance with a probe or primers specific for a toxin gene, only indicates that bacteria with those gene sequences are present and that they have the potential to be toxigenic. But, it does not indicate that the gene is actually expressed and that the toxin is made. Likewise, in clostridial and staphylococcal intoxication, DNA probes and PCR can detect only the presence of cells, but are of limited use in detecting the presence of preformed toxins (12).Currently, there are at least 30 assays each for testing for E. coli O157:H7 and for Salmonella. Such a large number of options can be confusing and overwhelming to the user, but, more importantly, has limited the effective evaluation of these methods. As a result, only few methods have been officially validated for use in food testing (1,11).ConclusionsAs a rapid method is used more frequently, its benefits and at the same time, its limitations also become more apparent. This section only briefly described some of the rapid method formats and selected problems encountered when using these assays in food analysis. However, because of the complex designs and formats of these tests, coupled with the difficulties of testing foods, users must exercise caution when selecting rapid methods and to also evaluate these tests thoroughly, as some may be more suitable than others for distinct testing situations or for assaying certain types of food. Lastly, technology continues to advance at a great pace and next generation assays, such as biosensors (18) and DNA chips (32) already are being developed that potentially have the capability for near real-time and on-line monitoring of multiple pathogens in foods.NOTE: The listings provided in Tables 1-4 are intended for general reference only and do not indicate endorsement or approval by FDA for use in food analysis.Table 1. Partial list of miniaturized biochemical kits and automated systems for identifyingfoodborne bacteria* ( 5, 8, 15, 16, 21, 35, 36 ).System Format Manufacturer OrganismsAPI b biochemical bioMerieux Enterobacteriaceae, Listeria, Staphylococcus,Campylobacter, Non-fermenters, anaerobes Cobas IDA biochemical HoffmannEnterobacteriaceaeLaRocheMicro-ID b biochemical REMEL Enterobacteriaceae, ListeriaEnterotubeII biochemical Roche EnterobacteriaceaeSpectrum 10 biochemical Austin Biological EnterobacteriaceaeRapID biochemical Innovative Diag. EnterobacteriaceaeBBL Crystal biochemical Becton Dickinson Enterobacteriaceae, Vibrionaceae, Non-fermenters,anaerobesMinitek biochemical Becton Dickinson EnterobacteriaceaeMicrobact biochemical Microgen Enterobacteriaceae, Gram negatives, Non-fermenters, ListeriaVitek b biochemical a bioMerieux Enterobacteriaceae, Gram negatives, GrampositivesMicrolog C oxidation a Biolog Enterobacteriaceae, Gram negatives, GrampositivesMIS b Fatty acid a Microbial-ID Enterobacteriaceae, Listeria, Bacillus,Staphylococcus, CampylobacterWalk/Away biochemical a MicroScan Enterobacteriaceae, Listeria, Bacillus,Staphylococcus, CampylobacterReplianalyzer biochemical a Oxoid Enterobacteriaceae, Listeria, Bacillus,Staphylococcus, CampylobacterRiboprinter nucleic acid a Qualicon Salmonella, Staphylococcus, Listeria,EscherichiacoliCobas Micro-ID biochemical a Becton Dickinson Enterobacteriaceae, Gram negatives, Non-fermentersMalthus b conductance a Malthus Salmonella, Listeria, Campylobacter, E. coli,Pseudomonas, coliformsBactometer impedance a bioMerieux Salmonella* Table modified from: Feng, P., App.I., FDA Bacteriological Analytical Manual, 8A ed.a Automated systemsb Selected systems adopted AOAC Official First or Final Action.NOTE: This table is intended for general reference only and lists known available methods. Presence on this list does not indicate verification, endorsement, or approval by FDA for use in food analysis.Table 2. Partial list of commercially-available, nucleic acid-based assays used in the detection of foodborne bacterial pathogens* (2, 5, 8, 12, 14, 22, 25, 36, 37).Organism Trade Name Format Manufacturer Clostridium botulinum Probelia PCR BioControlAccuProbe probe GEN-PROBE CampylobacterGENE-TRAK probe NeogenEscherichia coli GENE-TRAK probe NeogenBAX PCR a QualiconE. coli O157:H7Probelia PCR BioControlGENE-TRAK c probe NeogenListeriaAccuProbe probe GEN-PROBEBAX PCR QualiconProbelia PCR BioControlGENE-TRAK c probe NeogenSalmonellaBAX PCR QualiconBIND b phage BioControlProbelia PCR BioControlAccuProbe probe GEN-PROBE Staphylococcus aureusGENE-TRAK probe NeogenYersinia enterocolitica GENE-TRAK probe Neogen* Table modified from: Feng, P., App.I, FDA Bacteriological Analytical Manual, 8A ed.a Polymerase chain reactionb Bacterial Ice Nucleation Diagnosticsc Adopted AOAC Official First or Final ActionNOTE: This table is intended for general reference only and lists known available methods. Presence on this list does not indicate verification, endorsement, or approval by FDA for use in food analysis.Table 3. Partial list of commercially-available, antibody-based assays for the detection of foodborne pathogens and toxins* (3, 5, 8, 12, 33, 36).Organism/toxin Trade Name Assay Format a Manufacturer Bacillus cereus diarrhoealTECRA ELISA TECRAtoxinBCET RPLA UnipathCampyslide LA Becton Dickinson CampylobacterMeritec-campy LA MeridianMicroScreen LA MerciaVIDAS ELFA b bioMerieuxEiaFOSS ELISA b FossTECRA ELISA TECRA Clostridium botulinum toxin ELCA ELISA ElcatechC. perfringens enterotoxin PET RPLA Unipath Escherichia coliEHEC**c O157:H7RIM LA REMELE. coli O157 LA UnipathProlex LA PRO-LABEcolex O157 LA Orion DiagnosticaWellcolex O157 LA MurexE. coli O157 LA TechLabO157&H7 sera DifcoPetrifilmHEC Ab-blot 3MEZ COLI Tube-EIA DifcoDynabeads Ab-beads DynalEHEC-TEK ELISA Organon-TeknikaAssurance e ELISA BioControlHECO157 ELISA 3M CanadaTECRA ELISA TECRAE. coli O157 ELISA LMD LabPremier O157 ELISA MeridianE. coli O157:H7 ELISA BinaxE. coli Rapitest ELISA MicrogenTransia Card E. coli O157 ELISA DiffchambE. coli O157 EIA/capture TECRAVIP e Ab-ppt BioControlReveal Ab-ppt NeogenQuix Rapid O157 Ab-ppt Universal HealthWatchImmunoCardSTAT Ab-ppt MeridianVIDAS ELFA b bioMerieuxEiaFOSS ELISA b FossShiga toxin (Stx)VEROTEST ELISA MicroCarbPremier EHEC ELISA MeridianVerotox-F RPLA Denka SeikenETEC cLabile toxin (LT) VET-RPLA RPLA OxoidStabile toxin (ST) E. coli ST ELISA OxoidMicroscreen LA MicrogenListeriaListeria Latex LA MicrogenListeria-TEK e ELISA Organon TeknikaTECRA e ELISA TECRAAssurance e ELISA BioControlTransia Plate Listeria ELISA DiffchambPathalert ELISA MerckListertest Ab-beads VICAMDynabeads Ab-beads DynalVIP e Ab-ppt BioControlClearview Ab-ppt UnipathRAPIDTEST Ab-ppt UnipathVIDAS e ELFA b bioMerieuxEiaFOSS ELISA b FossUNIQUE Capture-EIA TECRABactigen LA Wampole Labs SalmonellaSpectate LA Rhone-PoulencMicroscreen LA MerciaWellcolex LA Laboratoire WellcomeSerobact LA REMELRAPIDTEST LA UnipathDynabeads Ab-beads DynalScreen Ab-beads VICAMCHECKPOINT Ab-blot KPL1-2 Test e diffusion BioControlSalmonellaTEK e ELISA Organon TeknikaTECRA e ELISA TECRAEQUATE ELISA BinaxBacTrace ELISA KPLLOCATE ELISA Rhone-PoulencAssurance e ELISA BioControlSalmonella ELISA GEM BiomedicalTransia Plate SalmonellaELISA DiffchambGoldBioline ELISA BiolineVIDAS e ELFA b bioMerieuxOPUS ELISA b TECRAPATH-STIK Ab-ppt LUMACReveal Ab-ppt NeogenClearview Ab-ppt UnipathUNIQUE e Capture-EIA TECRABactigen LA Wampole Labs ShigellaWellcolex Laboratoire WellcomeStaphyloslide LA Becton Dickinson Staphylococcus aureusAureusTest e LA TrisumStaph Latex LA DifcoS. aureus VIA ELISA TECRASET-EIA ELISA Toxin TechnologyenterotoxinSET-RPLA RPLA UnipathTECRA e ELISA TECRATransia Plate SE ELISA DiffchambRIDASCREEN ELISA R-BiopharmVIDAS ELFA b bioMerieuxOPUS ELISA b TECRAcholeraSMART Ab-ppt New HorizonVibrio cholerabengalSMART Ab-ppt New HorizoncholeraScreen Agglutination New HorizonbengalScreen Agglutination New Horizonenterotoxin VET-RPLA d RPLA Unipath* Table modified from: Feng, P., App.I, FDA Bacteriological Analytical Manual, 8A ed.a Abbreviations: ELISA, enzyme linked immunosorbent assay; ELFA, enzyme linked fluorescent assay; RPLA, reverse passive latex agglutination; LA, latex agglutination; Ab-ppt, immunoprecipitation.b Automated ELISAc EHEC - Enterohemorrhagic E. coli; ETEC - enterotoxigenic E. colid Also detects E. coli LT enterotoxine Adopted AOAC Official First or Final Action** CAUTION: unless the assays claim that they are specific for the O157:H7 serotype, most ofthese tests detect only the O157 antigen; hence will also react with O157 strains that are not of H7 serotype. These O157, non-H7 strains, generally do not produce Shiga toxins and are regarded as not pathogenic for humans. Furthermore, some antibodies to O157 can also cross react with Citrobacter, E. hermanii and other enteric organisms.NOTE: This table is intended for general reference only and lists known available methods. Presence on this list does not indicate verification, endorsement, or approval by FDA for use in food analysis.Table 4. Partial list of other commercially available rapid methods and specialty substrate media for detection of foodborne bacteria* (4, 8, 13, 20, 27, 36).Organism Trade Name Format a Assay Manufacturer BacteriaRedigel b Media RCR ScientificIsogrid b HGMF QA LabsEnliten ATP PromegaProfile-1 ATP New HorizonBiotrace ATP BiotraceLightning ATP IdexxPetrifilm b media-film 3MSim Plate media IdexxIsogrid b HGMF/MUG QA LabsColiform/ E. coliPetrifilm b media-film 3MSimPlate media IdexxRedigel Media RCR ScientificColiQuik c MUG/ONPG HachColiBlue c media HachColilert b,c MUG/ONPG IdexxLST-MUG b MPN media Difco & GIBCOColiComplete b MUG-Xgal BioControlColitrak MPN-MUG BioControlColiGel & E*Colite c MUG-Xgal Charm SciencesCHROMagar Medium CHROMagarMUG disc MUG REMELE. coliCHROMagar Medium CHROMagarEHEC dRainbow Agar Medium BiologBCMO157:H7 Medium BiosynthFluorocult O157:H7 Medium MerckListeria monocytogenes BCM Medium BiosynthIsogrid b HGMF QA LabsSalmonellaOSRT Medium/ motility Unipath (Oxoid)Rambach Medium CHROMagarMUCAP C8esterase BiolifeXLT-4 Medium DifcoMSRV b MediumYersinia Crystal violet Dye binding Polysciences* Table modified from: Feng, P., App.I, FDA Bacteriological Analytical Manual, 8A ed.a Abbreviations: APC, aerobic plate count; HGMF, hydrophobic grid membrane filter; ATP, adenosine triphosphate; MUG, 4-methylumbelliferyl-ß-D-glucuronide; ONPG, O-nitrophenyl ß-D-galactoside; MPN, most probable number.b Adopted AOAC Official First or Final Action.c Application for water analysisd EHEC - enterohemorrhagic Escherichia coliNOTE: This table is intended for general reference only and lists known available methods. Presence on this list does not indicate verification, endorsement, or approval by FDA for use in food analysis.References1. Andrews, W.H. 1996. AOAC INTERNATIONAL"S three validation programs for methods used inthe microbiological analysis of foods. Trend in Food Sci. Technol. 7:147-151.2. Barrett, T., P. Feng and B. Swaminathan. 1997. Amplification Methods for Detection ofFoodborne Pathogens, pp.171-181. In: H.H. Lee, S.A. Morse and O. Olsvik (ed), Nucleic AcidAmplification Techniques: Application to Disease Diagnosis. Eaton Publishing, Boston.3. Candish, A.A.G. 1991. Immunological methods in food microbiology. Food Microbiol. 8:1-14.4. Chain, V.S., and D.Y.C. Fung. 1991. Comparison of Redigel, Petrifilm, spiral plate system,Isogrid, and aerobic plate count for determining the numbers of aerobic bacteria in selectedfoods. J. Food Prot. 54:208-211.5. Cox, N.A., D.Y.C. Fung, J.S. Bailey, P.A. Hartman, and P.C. Vasavada. 1987. Miniaturized kits,immunoassays and DNA hybridization for recognition and identification of foodborne bacteria.Dairy Food Sanit. 7:628-631.6. Curiale, M.S., M.J. Klatt, and M.A. Mozola. 1990. Colorimetric deoxyribonucleic acid hybridizationassay for rapid screening of Salmonella in foods: Collaborative study. J. Assoc. Off. Anal. Chem.73:248-256.7. D'Aoust, J.-Y., A.M. Sewell, and P. Greco. 1991. Commercial latex agglutination kits for thedetection of foodborne Salmonella. J. Food Prot. 54:725-730.8. Dziezak, J.D. 1987. Rapid methods for microbiological analysis of foods. Food Technol. 41(7):56-73.9. Feldsine, P.T., R.L. Forgey, M.T. Falbo-Nelson and S. Brunelle.1997. Escherichia coli O157:H7Visual Immunoprecipitation assay: a comparative validation study. J. AOAC 80:43-48.10. Feng, P. 1992. Commercial assay systems for detecting foodborne Salmonella: a review. J. FoodProt. 55:927-934.11. Feng. P. 1996. Emergence of rapid methods for identifying microbial pathogens in foods. J.Assoc. Off. Anal. Chem. Int. 79:809-812.12. Feng, P. 1997. Impact of Molecular Biology on the Detection of Foodborne Pathogens. Mol.Biotech. 7:267-278.13. Feng, P.C.S., and P.A. Hartman. 1982. Fluorogenic assays for immediate confirmation ofEscherichia coli. Appl. Environ. Microbiol. 43:1320-1329.14. Feng, P., K.A. Lampel and W.E. Hill. 1996. Developments in food technology: applications andeconomic and regulatory considerations, pp. 203-229. In: C.A. Dangler and B. Osburn (ed).Nucleic Acid Analysis: Principles and Bioapplications. Wiley & Sons, New York,15. Fung, D.Y.C. 1991. Rapid methods and automation for food microbiology, pp. 1-38. In:Instrumental Methods for Quality Assurance in Foods. D.Y.C. Fung and R.F. Matthews (eds).Marcel Dekker, New York.16. Fung, D.Y.C., N.A. Cox, and J.S. Bailey. 1988. Rapid methods and automation in themicrobiological examination of food. Dairy Food Sanit. 8:292-296.17. Gaillot, O., P.D. Camillo, P. Berche, R. Courcol and C. Savage.1999. Comparison ofCHROMagar salmonella medium and Hektoen Enteric agar for isolation of salmonellae from stool samples. J. Clin. Microbiol. 37:762-765.18. Goldschmidt, M. 1999. Biosensors - scope in microbiological analysis, p. 268-278. In R.K.Robinson, C.A. Batt and P. Patel (ed.). Encyclopedia of Food Microbiology. Academic Press,London.19. Gutteridge, C.S., and M.L. Arnott. 1989. Rapid methods: an over the horizon view, pp. 297-319.In: Rapid Methods in Food Microbiology: Progress in Industrial Microbiology. M.R. Adams andC.F.A. Hope (eds). Elsevier, New York.20. Hartman, P.A. 1989. The MUG (glucuronidase) test for Escherichia coli in food and water, pp.290-308. In: Rapid Methods and Automation in Microbiology and Immunology. A. Balows, R.C.Tilton, and A. Turano (eds). Brixia Academic Press, Brescia, Italy.21. Hartman, P.A., B. Swaminathan, M.S. Curiale, R. Firstenberg-Eden, A.N. Sharpe, N.A. Cox,D.Y.C. Fung, and M.C. Goldschmidt. 1992. Chapter 39, Rapid methods and automation, pp. 665-746. In: Compendium of Methods for the Microbiological Examination of Foods, 3rd ed., C.Vanderzant and D.F. Splittstoesser (eds). American Public Health Association, Washington, DC.22. Hill, W.E. 1996. The polymerase chain reaction: application for the detection of foodbornepathogens. CRC Crit. Rev. Food Sci. Nutrit.36:123-173.23. Holmes, B. 1989. Comparative evaluation of the Roche Cobas IDA and Enterotube II systems foridentifying members of the family Enterobacteriaceae. J. Clin. Microbiol. 27:1027-1030.24. Ibrahim, G.F. 1986. A review of immunoassays and their application to salmonellae detection infoods. J. Food Prot. 49:299-310.25. Kalamaki, M., R.J. Prioce and D.Y.C. Fung. 1997. Rapid methods for identifying seafoodmicrobial pathogens and toxins. J. Rapid Methods and Automation in Microbiol. 5:87-137.26. Lampel, K.A., P. Feng and W.E. Hill.1992. Gene probes used in food microbiology, pp. 151-188.In D. Bhatnagar and T.E. Cleveland (ed.), Molecular Approaches to Improving Food Safety. Van Nostrand Reinhold, New York, NY.27. Lantz, P-G., B. Hahn-Hagerdal, and P. Radstrom. 1994. Sample preparation methods in PCR-based detection of food pathogens. Trend Food Sci. Technol. 5:384-389.28. Manafi, M., W. Kneifel and S. Bascomb. 1991. Flurogenic and chromogenic substrates used inbacterial diagnostics. Microbiol. Rev. 55:335-348.29. Miller, J.M., and D.L. Rhoden. 1991. Preliminary evaluation of Biolog, a carbon source utilizationmethod for bacterial identification. J. Clin. Microbiol. 29:1143-1147.30. Moberg, L.J., M.K. Wagner, and L.A. Kellen. 1988. Fluorogenic assay for rapid detection ofEscherichia coli in chilled and frozen foods: collaborative study. J. Assoc. Off. Anal. Chem.71:589-602.31. Oggel, J.J., D.C. Nundy, and C.J. Randall. 1990. Modified 1-2 test system as a rapid screeningmethod for detection of Salmonella in foods and feeds. J. Food Prot. 53:656-658.32. Olsvik, O., T. Popovic, E. Skjerve, K.S. Cudjoe, E. Hornes, J. Ugelstad and M. Uhlen. 1994.Magnetic separation techniques in diagnostic microbiology. Clin. Microbiol. Rev. 7:43-54.33. Ramsay, G.1998. DNA chips: State-of-the art. Nature Biotechnol. 16:40-44.34. Rose, S.A., and M.F. Stringer. 1989. Immunological methods, pp. 121-167. In: Rapid Methods inFood Microbiology: Progress in Industrial Microbiology. M.R. Adams and C.F.A. Hope (eds).Elsevier, New York.35. Rossen, L., P. Norskov, K. Holmstrom, O.F. Rasmussen. 1992. Inhibition of PCR by componentsof food samples, and DNA-extraction solutions. Int. J. Food Microbiol. 17:37-45.36. Stager, C.E., and J.R. Davis. 1992. Automated systems for identification of microorganisms. Clin.Microbiol. Rev. 5:302-327.37. Swaminathan, B. and P. Feng. 1994. Rapid Detection of Foodborne Pathogenic Bacteria. In:Annual Review of Microbiology, 48:401-426. Annual Reviews Inc., Palo Alto, CA.38. Tenover, F.C. 1988. Diagnostic deoxyribonucleic acid probes for infectious diseases. Clin.Microbiol. Rev. 1:82-101.39. Wolber, P.K. and R.L. Green. 1990. New rapid method for the detection of Salmonella in foods.Trends Food Sci. Technol.1:80-82.Hypertext Source: Bacteriological Analytical Manual, 8th Edition, Revision A, 1998. Revised 2000-July-18, Final Revision: 2001-Jan-25Author: Peter Feng。
有机合成发展及趋势
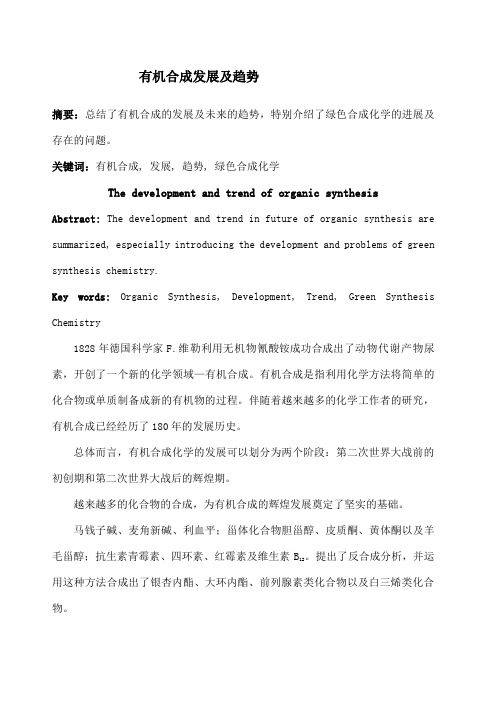
有机合成发展及趋势摘要:总结了有机合成的发展及未来的趋势,特别介绍了绿色合成化学的进展及存在的问题。
关键词:有机合成, 发展, 趋势, 绿色合成化学The development and trend of organic synthesisAbstract: The development and trend in future of organic synthesis are summarized, especially introducing the development and problems of green synthesis chemistry.Key words:Organic Synthesis, Development, Trend, Green Synthesis Chemistry1828年德国科学家F.维勒利用无机物氰酸铵成功合成出了动物代谢产物尿素,开创了一个新的化学领域—有机合成。
有机合成是指利用化学方法将简单的化合物或单质制备成新的有机物的过程。
伴随着越来越多的化学工作者的研究,有机合成已经经历了180年的发展历史。
总体而言,有机合成化学的发展可以划分为两个阶段:第二次世界大战前的初创期和第二次世界大战后的辉煌期。
越来越多的化合物的合成,为有机合成的辉煌发展奠定了坚实的基础。
马钱子碱、麦角新碱、利血平;甾体化合物胆甾醇、皮质酮、黄体酮以及羊毛甾醇;抗生素青霉素、四环素、红霉素及维生素B。
提出了反合成分析,并运12用这种方法合成出了银杏内酯、大环内酯、前列腺素类化合物以及白三烯类化合物。
如今,有机合成已经成为化学领域中最为重要的学科之一,其研究内容遍布材料、能源、环保、生命等各个学科,在社会文明发展与人们日常生活中发挥着极其重要的作用。
上世纪90年代,Seebach “Organic Synthesis-Where now?”的文章论述了当时的有机合成以及发展趋势[1],他认为从大的反应类型上讲有机合成已经难有新发现,当然新的改进和提高还在不断延续,而过渡金属参与的反应、对映和非对映的选择性反应以及在为的多步连续反应则可望成为以后发现新反应的领域。
有机分子在单晶金表面的自组装研究
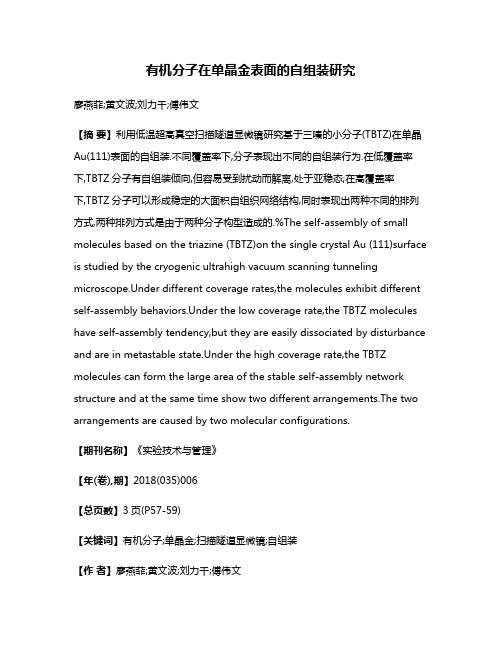
有机分子在单晶金表面的自组装研究廖燕菲;黄文波;刘力千;傅伟文【摘要】利用低温超高真空扫描隧道显微镜研究基于三嗪的小分子(TBTZ)在单晶Au(111)表面的自组装.不同覆盖率下,分子表现出不同的自组装行为.在低覆盖率下,TBTZ分子有自组装倾向,但容易受到扰动而解离,处于亚稳态;在高覆盖率下,TBTZ分子可以形成稳定的大面积自组织网络结构,同时表现出两种不同的排列方式,两种排列方式是由于两种分子构型造成的.%The self-assembly of small molecules based on the triazine (TBTZ)on the single crystal Au (111)surface is studied by the cryogenic ultrahigh vacuum scanning tunneling microscope.Under different coverage rates,the molecules exhibit different self-assembly behaviors.Under the low coverage rate,the TBTZ molecules have self-assembly tendency,but they are easily dissociated by disturbance and are in metastable state.Under the high coverage rate,the TBTZ molecules can form the large area of the stable self-assembly network structure and at the same time show two different arrangements.The two arrangements are caused by two molecular configurations.【期刊名称】《实验技术与管理》【年(卷),期】2018(035)006【总页数】3页(P57-59)【关键词】有机分子;单晶金;扫描隧道显微镜;自组装【作者】廖燕菲;黄文波;刘力千;傅伟文【作者单位】华南理工大学发光材料与器件国家重点实验室,广东广州 510641;华南理工大学发光材料与器件国家重点实验室,广东广州 510641;华南理工大学发光材料与器件国家重点实验室,广东广州 510641;华南理工大学材料科学与工程国家级实验教学示范中心,广东广州 510641【正文语种】中文【中图分类】TH742.9;O62有机光电子器件如有机发光二极管(OLED)[1-3]、有机太阳能电池(OPV)[4-6]、有机场效应晶体管(OFET)[7]等,因具有质轻、超薄、柔性、可溶液加工、易于大面积生产等诸多优势,被普遍认为是下一代光电子器件的发展方向。
- 1、下载文档前请自行甄别文档内容的完整性,平台不提供额外的编辑、内容补充、找答案等附加服务。
- 2、"仅部分预览"的文档,不可在线预览部分如存在完整性等问题,可反馈申请退款(可完整预览的文档不适用该条件!)。
- 3、如文档侵犯您的权益,请联系客服反馈,我们会尽快为您处理(人工客服工作时间:9:00-18:30)。
Recent Advances in Conjugated Polymer Energy StorageJared F.Mike,Jodie L.LutkenhausArtie McFerrin Department of Chemical Engineering,Texas A&M University,College Station,Texas77843 Correspondence to:J.L.Lutkenhaus(E-mail:jodie.lutkenhaus@)Received17December2012;revised9January2013;accepted11January2013;published online6February2013 DOI:10.1002/polb.23256ABSTRACT:This review covers recent advances in conjugated polymers and their application in energy storage.Conjugated polymers are promising cost-effective,lightweight,and flexi-ble electrode materials.The operating principles of conju-gated polymers are presented within the framework of their potential for energy storage.Special focus is given to polyaniline electrodes.Recent advances are reviewed includ-ing new methods of synthesis,nanostructuring,and assem-bly.Also,covered are applications that take full advantage of the mechanical properties of conjugated polymers and future applications of these novel materials.V C2013Wiley Periodicals,Inc.J.Polym.Sci.,Part B:Polym.Phys.2013,51, 468–480KEYWORDS:batteries;conducting polymers;conjugated poly-mers;energy;energy storage;flexible battery;flexible superca-pacitor;polyaniline;polymer battery;pseudocapacitor; supercapacitorsINTRODUCTION Storage of energy through electrochemical means(batteries,supercapacitors)is critical to providing portable power for technical applications such as laptops, smartphones,electric vehicles,military field electronics, pacemakers,glucose sensors,and many other devices.‘‘Plas-tic power,’’or energy and power sources consisting entirely (or mostly)of polymeric materials,offers several exciting opportunities for portable power.For example,a polymeric power source is potentially flexible,lightweight,moldable or castable,and multifunctional.This review will focus on the growing potential of conjugated polymers as electrodes for plastic power and recent advances occurring within the last 5years of the field.A particular focus will be placed on non-aqueous systems,which can achieve higher potentials. Conjugated polymers offer not only a means to store energy but also a means to do so independent of form factor.The mechanical properties of conjugated polymers are similar to those of a plastic,allowing them to be bent,flexed,or even stretched.1–8Without the need for a traditional prismatic or cylindrical cell,conjugated polymeric electrodes could be incorporated seamlessly into textiles,data tattoos,electronic paper,structural panels,and so forth.8–14Additionally,it is advantageous to directly combine plastic power with poly-mer solar cells or light emitting diodes in a streamlined manufacturing process to create low-cost fully flexible devices.10,15,16Aside from their mechanical versatility,conjugated polymers have several advantages such as synthetic tailorability,cost, and processability that make them ideal candidates for elec-trochemical energy storage.Their electrochemical and physi-cal properties may be tuned through organic synthetic tech-niques.1,17,18Furthermore,the raw materials and methods to produce these polymers are often economical.New synthetic approaches have enhanced processability such that conju-gated polymers can be processed via screen printing,doctor blading,or inkjet printing.19–21Other advantages include electrical conductivity and high coulombic efficiency with some polymers able to be cycled hundreds to thousands of times with little degradation.18,22,29In light of this,conju-gated polymers are ideal materials for hybrid composites in that they work synergistically with inorganic compounds that have high capacity but lack the conductivity and cyclability necessary for commercial application.23–26The combination of synthetic tuning,processing options,and me-chanical flexibility results in plastic power sources that can be designed to suit the device rather than vice versa.Most research in conjugated polymer electrodes for energy storage has focused on three polymers—polyaniline(PANI), polypyrrole,and polythiophene(PT)—and derivatives thereof,Figure1.18,27,28The focus has lain on these particu-lar polymers because of the presence of extensive back-ground research,the commercial availability of both mono-mers and polymers,and their ease of synthesis.The structures in Figure1are only a representative sample, whereas Nov a k’s1997review presents a more exhaustive list of structural variety.Of particular note is PANI,which was once a cathode in a PANI|lithium battery commercially available from Bridgestone-Seiko in the late1980s.29Table1 presents a comparison between common battery materials, LiCoO2and LiFePO4,30,31and the conjugated polymers pre-sented in Figure1.V C2013Wiley Periodicals,Inc.468JOURNAL OF POLYMER SCIENCE,PART B:POLYMER PHYSICS2013,51,468–480FEATURE ARTICLE JOURNAL OF POLYMERSCIENCEAlthough there are clear advantages,there are several issues that must be addressed before the full potential of conju-gated polymers can be realized.These include oxidative sta-bility,diffusion limitations of ions of dopants,low energy and power density,and low capacity.Several recent advances in the field have made significant headway in addressing these issues,and will be discussed in this review.OPERATING PRINCIPLESTo understand the operation of conjugated polymers within the framework of a battery electrode,we must first consider the fundamental properties of conjugated polymers them-selves.The conductivity and charge storage capabilities of conjugated polymers depend on electronic properties not possessed by common aliphatic polymers.The origin of interesting electronic and optical properties of conjugated polymers comes from the overlap of adjacent p -orbitals.The combination of molecular orbitals along the backbone of a conjugated polymer gives rise to a band structure analogous to that seen in inorganic semiconductors.37,38The effective conjugation length is the point at which no additional mono-mer units added to the chain affect the electronic propertiesof the polymer.39–41For organic semiconductors,the bottom of the conduction band is referred to as the lowest unoccu-pied molecular orbital (LUMO),while the top of the valence band is referred to as the highest occupied molecular orbital (HOMO).The values of the HOMO and LUMO energy levels determine properties such as oxidative stability,optical absorption wavelengths,and whether a polymer is more likely to be n-type or p-type.26,42Polymers can be classified as being either n-type or p-type based on whether they are easily reduced or oxidized,respec-tively.Electron rich structures that have higher HOMO levels make good p-type materials due to their ability to stabilize a positive charge,while electron poor structures are able to sta-bilize negative charges in low-lying LUMO levels,making them good n-type materials.43–45Generally speaking,n-doped poly-mers act as anodes,while p-doped polymers function well in cathodes.18There has been much work on p-type materials for cathodes,but there has been little focus on the synthesis of new n-type materials.46–48For fully flexible polymer batteries to be realized,both electrodes should be plastic,and so there is a need to research new n-type electrode materials as well.Conjugated polymers can be doped through either chemical or electrochemical means.49–51Figure 2illustrates this process for PT ,which can be p-doped or n-doped.Doping creates radi-cal cations or anions,also referred to as positive or negative polarons,respectively,which are delocalized along the poly-mer backbone and are a proposed mechanism for conductiv-ity.49,52,53Charge delocalization is coupled to structural reor-ganization to a quinoidal form and alters fundamental molecular vibrations.52The appearance of new subgap energy states and formation of quinoidal structure can be observed as changes in the UV/vis and IR/Raman spectra of poly-mers.49,52–56Conjugated polymers in their native state have very low conductivities,on the order of 10À4–10À9S/cm,but can attain very high conductivities when doped.38,57Poly(3,4-ethylenedioxythiophene)(PEDOT),for example,has exhibited conductivities as high as 1500S/cm in certain cases.58Jodie L.Lutkenhaus is an Assistant Professor of Chemical Engineering at Texas A&M University.Research specialties include polymeric electrodes for energy storage,polyelec-trolytes,ultrathin films and nanostructured polymers,as well as quartz crystal microbalance with dissipation.She received her B.S.in Chemical Engineering from The University of Texas at Austin in 2002and her Ph.D.in Chemical Engineering from the Massachusetts Institute of Technology in 2007.She received the NSF CAREER Award and ACS PRF-DNI in2011.Jared F.Mike received his B.S.from Youngstown State University in 2005and Ph.D.from Iowa State University in 2011with Professor Malika Jeffries-EL.Since 2011,he has been a postdoctoral researcher in Dr.Jodie Lutkenhaus’s laboratory at Texas A&M University.He is currently developing new conjugated polymers and studying their electrochemical performance as energy storage materials.He is also studying the effects of functionalization on the electrochemical properties of conjugatedpolymers.FIGURE 1Examples of common conjugated polymers found in energy storage research.JOURNAL OF POLYMERSCIENCEFEATUREARTICLEJOURNAL OF POLYMER SCIENCE,PART B:POLYMER PHYSICS 2013,51,468–480469To maintain charge balance,redox reactions associated with polymer doping are concurrent with the movement of ions into and out of the polymer matrix.59,60Although oxidation generally results in anion movement within the film,studies have shown that under certain conditions,cation movement occurs as well.61–63It could be inferred that analogous processes can also occur for anions during polymer reduc-tion.As polymer films grow thicker,they tend to respond more slowly due to ion transport limitations,giving rise to lower capacities as well as energy and power den-sities.6,18,64,65Polymer nanostructures such as nanowires,nanotubes,or nanoparticles can help to alleviate problems associated with ion diffusion by increasing the surface area and porosity.66–68TABLE 1Performance Characteristics of Conjugated Polymers as Compared to Current Inorganic Li-Ion Battery MaterialsCathode Operating Voltage (V vs.Li/Li þ)MW of repeat unit Theoretical Capacity a (mAh/g)Experimental Capacity (mAh/g)Energy Density (mWh/g)PowerDensity (mW/g)LiCoO 230$3.897.8727413530–144100–1,000LiFePO 431$3.2157.7617010990–10023–70Polyaniline 233–491.11294100–147300100Polypyrrole 1,323–465.074128260$10Polythiophene 33,34 3.1–482.12326829389PEDOT 35,36$2.7–4.2140.1619130–701–435–2,500aBased on a doping level of 1electron per monomerunit.FIGURE 2Illustration of PT electronic and chemical structural changes during a)oxidation (p-doping)and b)reduction (n-doping).A radical cation (positive polaron)is formed from oxidation,while a radical anion (negative polaron)is formed during reduction.High states of charging can lead to the formation of positive and negative bipolarons.Cations (C þ)and anions (A À)counterbal-ance charges in the polymer chain.FEATURE ARTICLEJOURNAL OF POLYMERSCIENCE470JOURNAL OF POLYMER SCIENCE,PART B:POLYMER PHYSICS 2013,51,468–480Besides ion diffusion,oxidative stability also affects the elec-trode’s performance.The number of monomer units over which a charge is delocalized determines the specific capacity of the electrode.For most conjugated polymers,this amounts to one unit of charge for every two to three mono-mer units,18,60although higher levels of charging are possi-ble.A higher specific capacity is realized with higher levels of oxidation or reduction.Unfortunately,high levels of charg-ing can lead to destabilization of the polymer matrix through unwanted interchain interactions or breakdown of polymer chains.69,70Additionally,highly charged species are much more likely to react with common electrolytes and/or oxygen in the air.69,71,72Low capacities can be mitigated to a limited extent through choice of dopant ion,electrolyte,or polymer structure.69,73–78There is evidence that strong interactions between the highly charged or doped conjugated polymer and a complementary polyion can serve to stabilize highly oxidized conjugated polymers.79Deficiencies associated with oxidative stability over many charge cycles have seen improvements with select composite materials such as gra-phene or carbon nanotubes.1,80–83SYNTHESISSynthesis of conjugated polymers for electrodes may be accomplished through electrochemical or chemical means.In general,oxidation is used to provide the driving force for polymer synthesis,whether it is through the addition of a chemical oxidant(e.g.,ammonium persulfate)or through the application of an oxidizing electrochemical potential to the surface of a submerged electrode.60As this type of synthesis is performed in an oxidizing environment,the resulting poly-mers are formed in an already doped state.PANI,for exam-ple,is synthesized in the emeraldine salt state under oxida-tive conditions.Reductive polymerization is not unheard of, but is rarely employed primarily due to limited applicabil-ity.84–87This is possibly one reason p-type polymers are more commonly studied than n-type polymers.Conjugated polymers are most often synthesized in a solution of mono-mer and electrolyte,but there are examples of solid-state or vapor deposition polymerizations as well.58,88,89Metal-cata-lyzed cross-couplings are another method for producing con-jugated polymers.90–94Unlike oxidative synthesis,polymers synthesized in this way are formed in a charge-neutral state. Unfortunately,these systems can sometimes require the presence of specific functional groups and sensitive reaction conditions not conducive to producing large quantities of material suitable for electrodes.However,promising advan-ces in direct arylation polymerizations are working toward eliminating the need for generating monomers with specific functional group requirements.95Metal-catalyzed polycon-densations are also a potential method for synthesizing new n-type or specially functionalized materials.90,96Several recent synthetic approaches have focused upon increasing the solubility or dispersibility of conjugated poly-mers to enhance their processability.Side chains are essen-tial for solubilizing conjugated polymers used in semicon-ducting applications,and similar concepts are applicable for electrochemical energy storage.97–100Side chain functionali-zation has been used to facilitate intimate mixing in compo-sites.32,101–103Conjugated polymer:polyelectrolyte complexes have also come a long way to improve polymer process-ing.104–106For example,poly(2-acrylamido-2-methyl-1-pro-panesulfonic acid)has been shown to both solubilize and stabilize PANI in complexes.79,107–109PANIPANI|lithium batteries are one of few examples of polymer-based energy storage to have been commercialized.Research interest in PANI continues to grow due to its oxidative stabil-ity,relatively high theoretical capacity,simple synthesis,and electrochemical reversibility.30,110PANI is an interesting case with its many redox states,111–113(Fig.3).Only emeraldine salt is conductive,although the conductivity of pernigraniline salt is still not entirely clear,and more recent reports suggest that it is.114–117PANI electrodes make use of the reversible reactions between fully reduced leucoe-meraldine base and partially oxidized emeraldine salt.At higher potentials,cycling stability can break down as emeral-dine salt irreversibly oxidizes to pernigraniline base23 because the reaction pathway to pernigraniline salt is not favored.There have been efforts to stabilize the pernigrani-line salt,79,116,117which allows for the doubling of PANI’s capacity and increasing its energy density.Although there are many advantages,problems associated with the utilization of PANI stem from insolubility,diffusion issues,cycling,and stability.Insolubility and intractability of PANI are often considered to be the main issues hindering its usage in energy storage.118These problems had tradition-ally been addressed through dispersing the polymer in solu-tions using a series of steps intended to remove aggregates and ensure a stable suspension.119More recently,template synthesis using polyanions and the synthesis of PANI nano-structures,most notably nanofibers,has enabled easy solu-tion suspension for PANI.67,118,120Cycling stability has been addressed by combining PANI with carbon materials such as graphene or carbon nanotubes.Carbonaceous materials store charge through non-faradaic reactions and possess high cy-cling stability.They help to maintain conductivity in a com-posite when PANI has been reduced to a non-conductive state.Therefore,composites of PANI and carbon materials are promising candidates for energy storage.1,46,80,83Among the many varieties of synthesizable nanostructures for conjugated polymers,there is particular interest in one-dimesional nanostructures such as nanotubes,nanorods,or nanofibers.121,122PANI nanofibers(PANI-NF)are especially attractive since they can be made economically and quickly in solution without the use of templates,additives,or compli-cated synthetic techniques.122Kaner and coworkers have developed synthetic methods for variable diameter PANI-NF that involve either rapidmixing of oxidant and monomer solu-tions or interfacial polymerization in phase-separated solvent systems(Fig.4).67,123–125The size of the nanofibers can be controlled by choice of reactant medium and acid.77,108,109JOURNAL OF POLYMERSCIENCE FEATUREARTICLE JOURNAL OF POLYMER SCIENCE,PART B:POLYMER PHYSICS2013,51,468–480471As expected,PANI-NFs show improved electrochemical per-formance over conventional solution-synthesized PANI (Table 2).126–128Perhaps the most attractive feature of PANI-NFs,however,is their ability overcome intractability issues because they are easily suspended in aqueous solution and remain so for extended periods of time.67As previously stated,nanostructured carbon materials such as graphene,carbon nanotubes,or even carbon black are de-sirable for their high conductivity and stability,147–150yet can suffer from lower capacity versus redox active materials due to the non-Faradaic nature of charge storage.80,151,152PANI is an ideal material for polymer/carbon composites because of its cyclability,electrochemical activity,and so forth;several examples are highlighted in Table 2.Carbonnanotubes or graphene/PANI composite electrodes are usu-ally formed by pressing into pellets,vacuum-assisted filtra-tion,or by polymerization on carbon nanotube surfaces or films,for example.130,131,133–135,139–144,146–158Although these electrodes can have good electrochemical characteristics,there are drawbacks to their fabrication such as requiring additional pre-or post-treatment of samples or special ex-perimental conditions to form a workingcathode.133,137,138,145,158The processability of PANI-NFs allows for the facile solution synthesis of composite electrodes.Hammond’s group has demonstrated the successful layer-by-layer assembly of PAN-I:multiwalled carbon nanotube films (Fig.5).159The result-ing film was highly porous,and was able to overcomeionFIGURE 4Transmission electron microscope (TEM)images of PANI-NF formed through (a)interfacial and (b)rapid mixing poly-merization (Reproduced from Refs.124and 125,with permission VC 2002American Chemical Society and V C 2004John Wiley &Sons,Inc.)FIGURE 3Illustration of the redox states of PANI and the chemical reactions associated with their interconversion.FEATURE ARTICLEJOURNAL OF POLYMERSCIENCE472JOURNAL OF POLYMER SCIENCE,PART B:POLYMER PHYSICS 2013,51,468–480T A B L E 2S e l e c t e d P o l y a n i l i n e C o m p o s i t e s w i t h G r a p h e n e o r S i n g l e -W a l l e d o r M u l t i -W a l l e d C a r b o n N a n o t u b e sC a t h o d e S p e c i f i c C a p a c i t a n c e (F /g )C h a r g i n g R a t e aE l e c t r o l y t eE n e r g y D e n s i t y (m W h /g )P o w e r D e n s i t y (m W /g )bN o t e sP A N I 12733c1M C a m p h o r s u l f o n i c a c i dB u l k P A N I s y n t h e s i z e d b y c h e m i c a l m e t h o dP A N I -N F 127122c1M C a m p h o r s u l f o n i c a c i dC h e m i c a l s y n t h e s i s o f P A N I n a n o f i b e r s u s i n g n a n o f i b e r s s e e d sP A N I -N F 129554d1.0A /g1M H 2S O 4I n t e r f a c i a l p o l y m e r i z a t i o nP A N I /P o r o u s C a r b o n1,270–2,200c66.7-.67A /g1M H 2S O 4173–30047,000–70E l e c t r o d e p o s i t i o n o f P A N I o n t o h i e r a r c h i c a l l y p o r o u s c a r b o n m o n o l i t hP A N I /P o r o u s C a r b o n768–900c5–0.5A /g1M H 2S O 4E l e c t r o d e p o s i t i o n o f P A N I o n t o m e s o p o r o u s c a r b o n m o n o l i t hP A N I /S W N T 130485c0.01A /c m 21M H 2S O 4120–2282,250–520E l e c t r o d e p o s i t i o n o f P A N I o n t o S W N T /b i n d e r f i l m o n s t a i n l e s s s t e e l s h e e tP A N I /S W N T 131236d10A /g1M L i C l O 41:1:1E C /D E C /D M C13162500E l e c t r o d e p o s i t e d P A N I o n f r e e -s t a n d i n g S W N T f i l mP A N I -N F /M W N T132417d1m A /c m21M L i P F 63:7E C :D M C$100–500300,000–300D i p p e d l a y e r -b y -l a y e r s o l u t i o n a s s e m b l y o n I T OP A N I /M W N T133360–424d1.0–0.2A /g1M H 2S O 46–10C h e m i c a l s y n t h e s i s o f P A N I o n ‘‘B u c k y P a p e r ’’m a d e b y f i l t r a t i o n o f M W N T sP A N I /M W N T 134650,c 360d$0.2A /g1M H 2S O 4C h e m i c a l s y n t h e s i s o n t o M W N T s i n s o l u t i o n ,p r e s s e d i n t o p e l l e tP A N I /C N T 135978c118A /g1M H 2S O 4E l e c t r o d e p o s i t e d P A N I o n v e r t i c a l l y o r i e n t e d c a r b o n n a n o t u b e a r r a yP A N I /M W N T 136332d1.0A /g S o l i d s t a t e H 2S O 4ÀP V A g e l7.12,189C h e m i c a l s y n t h e s i s o n t o ‘‘B u c k y P a p e r ’’P A N I /G r a p h e n e 137170(F /c m 3)c100A /c m 31M N a 2S O 4D i p p e d l a y e r -b y -l a y e r s o l u t i o n a s s e m b l y o n I T OP A N I /G r a p h e n e 138116–375c3.0–0.5A /g1M H 2S O 430.341,000D i p p e d l a y e r -b y -l a y e r s o l u t i o n a s s e m b l y o n I T OP A N I /G r a p h e n e 1391046c1m V /sQ 6M K O H39–14570,000–522C h e m i c a l s y n t h e s i s i n a s u s p e n s i o n o f g r a p h e n e o x i d e ,m i x e d w i t h b i n d e r s a n d c o a t e d o n t o N i f o a mP A N I -N F /G r a p h e n e 140531c0.2A /g1M H 2S O 4C h e m i c a l s y n t h e s i s i n a s u s p e n s i o n o f g r a p h e n e o x i d e ,m i x e d w i t h b i n d e r s a n d p r e s s e d o n t o S S s h e e tP A N I -N F /G r a p h e n e 141197–210d3.0–0.3A /g1M H 2S O 4M i x e d s u s p e n s i o n o f P A N I a n d g r a p h e n e f i l t e r e d t o p r o d u c e a f i l mP A N I -N F /G r a p h e n e 142321–526c1.0–0.2A /g2M H 2S O 4C h e m i c a l s y n t h e s i s i n a s u r f a c t a n t -s t a b i l i z e d s u s p e n s i o n o f g r a p h e n e o x i d e ,d r o p c a s t o n t o g l a s s y c a r b o n e l e c t r o d eP A N I N a n o w i r e s /G r a p h e n e 143227–555d2.0–0.2A /g1M H 2S O 4C h e m i c a l s y n t h e s i s o f P A N I n a n o w i r e s o n g r a p h e n e ,m i x e d w i t h b i n d e r ,p r e s s e d o n t o g o l d g r i dP A N I -g -G r a p h e n e 144250c1M H 2S O 4C h e m i c a l s y n t h e s i s o f P A N I g r a f t e d f r o m g r a p h e n e s h e e t s ,d r o p c a s t o n t o g l a s s y c a r b o n e l e c t r o d eP A N I N a n o r o d /G r a p h e n e 145974d2.5A /gH 3P O 4-P V A g e lC h e m i c a l s y n t h e s i s o n t o g r a p h e n e p a t t e r n e d b y m i c r o m o l d i n g i n c a p i l l a r i e sP A N I /G r a p h e n e /C N T 146341–569c10–0.1A /g1M H C l C h e m i c a l s y n t h e s i s o f P A N I o n C N T s ,s u s p e n d e d w i t h g r a p h e n e ,p r e s s e d o n t o c o n d u c t i v e t a p e o n P ta ,bF o r v a l u e s g i v e n a s a r a n g e ,t h e h i g h v a l u e f o r t h e c a p a c i t a n c e o r e n e r g y c o r r e s p o n d s t o t h e l o w v a l u e f o r c h a r g i n g r a t e s o r p o w e r .cT h r e e -e l e c t r o d e m e a s u r e m e n t .d T w o -e l e c t r o d e m e a s u r e m e n t .JOURNAL OF POLYMERSCIENCEFEATUREARTICLEJOURNAL OF POLYMER SCIENCE,PART B:POLYMER PHYSICS 2013,51,468–480473diffusion limitations at high rates of discharge.Several groups have also produced similar PANI/graphene films using layer-by-layer deposition methods.137,138,159Layer-by-layer films were formed by alternately immersing a surface-treated ITO-coated glass substrate into separate solutions of positively charged PANI-NFs and negatively charged func-tionalized carbon nanotubes or graphene.132,137,138,160,161Electrostatic interactions and entropic contributions enabled film formation at the surface.162This technique is advanta-geous in that it allows for the solution fabrication of intimate composites without the need for binders.In addition,layer-by-layer film formation is versatile and can be performed on many different surfaces such as textiles,walls,wood,glass,metal,and so forth.163,164With the advent of spray layer-by-layer assembly,165composites such as these could be rapidly manufactured on an industrial scale onto many substrates or even applied as paint.9,163–166PANI is also a promising material for composites with high capacity inorganic materials that lack ionic and electronic conductivity.23,167,168Our group has recently combined PANIand vanadium pentoxide in a layer-by-layer electrode (Fig.6).23V 2O 5is a high capacity metal oxide that shows promise for battery applications but suffers from poor elec-tronic conductivity and slow lithium ion diffusion.169,170By combining it with PANI,a composite can be formed that func-tions better than either material alone.23,171,172Motivated by the work of the Oliveira group,171,173we recently identified assembly conditions that form high capacity,energy dense cathodes.23,174By judiciously selecting the pH of assembly,the cycle thickness was dramatically increased,resulting in a shortened processing time.Overall,investigations of V 2O 5/PANI composites have demonstrated promise because the two components work together synergistically to improve coulombic efficiency and charge transport.23,171,172,175–177Our work has shown that the capacity is optimized at a par-ticular thickness,where thicker films are subject to ion diffu-sion limitations.23To address this issue,we are replacing conventionally synthesized PANI with PANI-NF to introduce a porous electrode morphology.174Other metal oxides and inorganics have also been successfully combined with PANI to produce synergistic composites.24,168,178–189FIGURE 5Illustration of the layer-by-layer assembly of PANI/carbon nanotube films (Reproduced from Ref.133,with permission VC 2011American ChemicalSociety.)FIGURE 6Illustration describing the steps of the layer-by-layer assembly process as applied to PANI/vanadium pentoxide compo-sites on ITO slides (Reproduced from Ref.21,with permission VC 2011American Chemical Society.)FEATURE ARTICLEJOURNAL OF POLYMERSCIENCE474JOURNAL OF POLYMER SCIENCE,PART B:POLYMER PHYSICS 2013,51,468–480。