Membrane bioreactor (MBR) for wastewater treatment
膜生物反应器原理结构
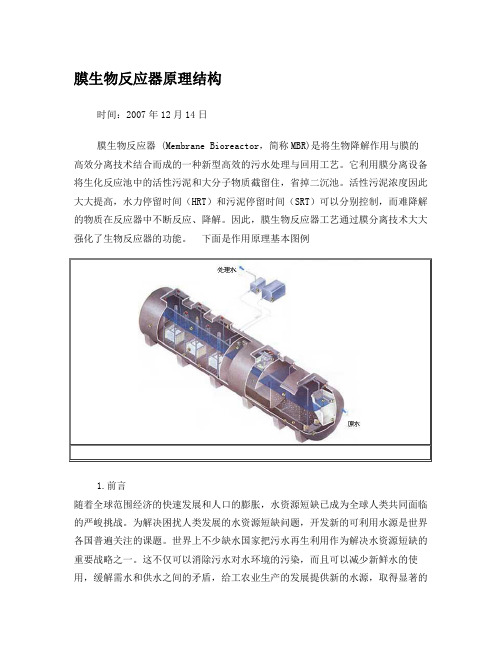
膜生物反应器原理结构时间:2007年12月14日膜生物反应器 (Membrane Bioreactor,简称MBR)是将生物降解作用与膜的高效分离技术结合而成的一种新型高效的污水处理与回用工艺。
它利用膜分离设备将生化反应池中的活性污泥和大分子物质截留住,省掉二沉池。
活性污泥浓度因此大大提高,水力停留时间(HRT)和污泥停留时间(SRT)可以分别控制,而难降解的物质在反应器中不断反应、降解。
因此,膜生物反应器工艺通过膜分离技术大大强化了生物反应器的功能。
下面是作用原理基本图例1.前言随着全球范围经济的快速发展和人口的膨胀,水资源短缺已成为全球人类共同面临的严峻挑战。
为解决困扰人类发展的水资源短缺问题,开发新的可利用水源是世界各国普遍关注的课题。
世界上不少缺水国家把污水再生利用作为解决水资源短缺的重要战略之一。
这不仅可以消除污水对水环境的污染,而且可以减少新鲜水的使用,缓解需水和供水之间的矛盾,给工农业生产的发展提供新的水源,取得显著的环境、经济和社会效益。
开展新型高效污水处理与回用技术的研究对于推进污水资源化的进程具有十分重要的意义。
膜-生物反应器是近年新开发的污水处理与回用技术。
该技术由于具有诸多传统污水处理工艺所无法比拟的优点,在世界范围受到普遍关注。
本文将对近年来膜-生物反应器污水处理与回用技术的研究与应用进行介绍。
2.膜-生物反应器的技术原理与特点在膜-生物反应器中,由于用膜组件代替传统活性污泥工艺中的二沉池,可以进行高效的固液分离,克服了传统活性污泥工艺中出水水质不够稳定、污泥容易膨胀等不足,从而具有下列优点[1]:(1)能高效地进行固液分离,出水水质良好且稳定,可以直接回用;(2)由于膜的高效截留作用,可使微生物完全截留在生物反应器内,实现反应器水力停留时间(HRT)和污泥龄(SRT)的完全分离,使运行控制更加灵活稳定;(3)生物反应器内能维持高浓度的微生物量,处理装置容积负荷高,占地面积省;......膜生物反应器(MBR)是一种由膜过滤取代传统生化处理技术中二次沉淀池和砂滤池的水处理技术。
血液制品污水处理MBR工艺使用及维护

血液制品污水处理MBR工艺使用及维护血液制品生产过程中产生的废水含有高浓度的有机物和微生物,对环境造成严重污染。
为了有效地处理这些废水,血液制品污水处理一般采用膜生物反应器 (membrane bioreactor, MBR) 工艺。
MBR工艺是一种结合了传统污水处理工艺和膜技术的处理方式,其主要目的是去除废水中的有机物和微生物,使出水满足排放标准。
MBR工艺的关键部分是膜组件,通常有微孔滤膜和超滤膜两种。
MBR工艺的处理过程包括生化反应和固液分离两个阶段。
废水进入生物反应器,通过微生物的降解作用,有机物被转化为无机物。
在这个过程中,微生物附着在膜组件表面,形成一层活性污泥膜。
然后,废水经过膜组件的筛选和阻截作用,固液分离出生物反应器中的悬浮物,产生清澈的处理水。
MBR工艺相比传统的活性污泥法具有以下几个显著优点:1. 出水水质稳定:MBR工艺可以有效地去除废水中的有机物和微生物,出水水质稳定,基本达到排放标准。
2. 占地面积小:MBR工艺通常采用高浓缩污泥处理方式,可以大幅度减小污泥产生量,减小处理设备的占地面积。
3. 运行稳定可靠:MBR工艺采用膜分离技术,具有良好的固液分离效果,膜组件的抗污染能力强,能够稳定运行长时间。
4. 适应性强:MBR工艺适用于大部分血液制品生产厂的废水特性,可以根据实际情况进行工艺调整,使其适应不同的废水处理要求。
MBR工艺的使用和维护也面临一些问题:1. 膜堵塞:由于废水中含有较高浓度的有机物和微生物,易导致膜组件堵塞。
解决这个问题需要进行定期的清洗和维护,保持膜组件的通量。
2. 膜污染:废水中的有机物和微生物附着在膜组件表面,形成污染物膜,会导致膜的渗透性能下降。
膜污染可以通过物理清洗、化学清洗和生物清洗等方式进行处理。
3. 运行能耗高:MBR工艺需要提供较高的跨膜压力才能保持膜组件的正常运行,因此需要消耗较大的能量。
为了降低能耗,可以采用一些节能措施,如控制通气量和调整水质参数等。
膜生物反应器原理结构
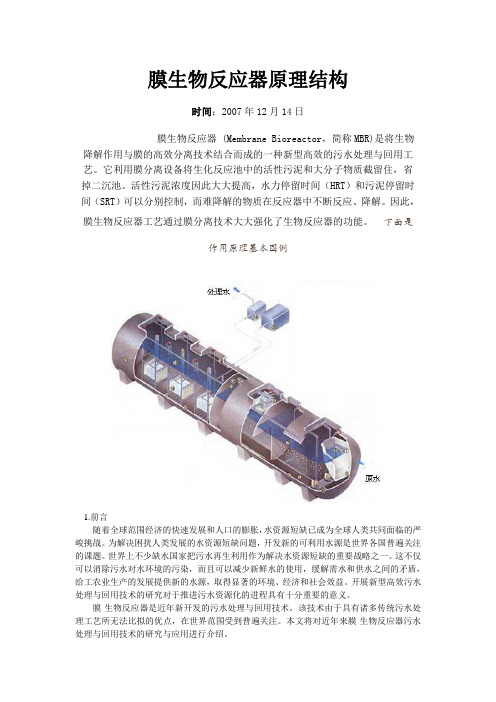
膜生物反应器原理结构时间:2007年12月14日膜生物反应器 (Membrane Bioreactor,简称MBR)是将生物降解作用与膜的高效分离技术结合而成的一种新型高效的污水处理与回用工艺。
它利用膜分离设备将生化反应池中的活性污泥和大分子物质截留住,省掉二沉池。
活性污泥浓度因此大大提高,水力停留时间(HRT)和污泥停留时间(SRT)可以分别控制,而难降解的物质在反应器中不断反应、降解。
因此,膜生物反应器工艺通过膜分离技术大大强化了生物反应器的功能。
下面是作用原理基本图例1.前言随着全球范围经济的快速发展和人口的膨胀,水资源短缺已成为全球人类共同面临的严峻挑战。
为解决困扰人类发展的水资源短缺问题,开发新的可利用水源是世界各国普遍关注的课题。
世界上不少缺水国家把污水再生利用作为解决水资源短缺的重要战略之一。
这不仅可以消除污水对水环境的污染,而且可以减少新鲜水的使用,缓解需水和供水之间的矛盾,给工农业生产的发展提供新的水源,取得显著的环境、经济和社会效益。
开展新型高效污水处理与回用技术的研究对于推进污水资源化的进程具有十分重要的意义。
膜-生物反应器是近年新开发的污水处理与回用技术。
该技术由于具有诸多传统污水处理工艺所无法比拟的优点,在世界范围受到普遍关注。
本文将对近年来膜-生物反应器污水处理与回用技术的研究与应用进行介绍。
2.膜-生物反应器的技术原理与特点在膜-生物反应器中,由于用膜组件代替传统活性污泥工艺中的二沉池,可以进行高效的固液分离,克服了传统活性污泥工艺中出水水质不够稳定、污泥容易膨胀等不足,从而具有下列优点[1]:(1)能高效地进行固液分离,出水水质良好且稳定,可以直接回用;(2)由于膜的高效截留作用,可使微生物完全截留在生物反应器内,实现反应器水力停留时间(HRT)和污泥龄(SRT)的完全分离,使运行控制更加灵活稳定;(3)生物反应器内能维持高浓度的微生物量,处理装置容积负荷高,占地面积省;......MBR膜生物反应器2003-06-17技术概况·由于采用了先进的膜生物反应器技术,使系统出水水质在各个方面均优于传统的污水处理设备,出水水质在感官上已接近于自来水的情况,可以作为中水回用。
【技术】浸没式膜生物反应器(MBR)(二)原理、滤膜、型式详解(污水设计、培训及购买必备知识)
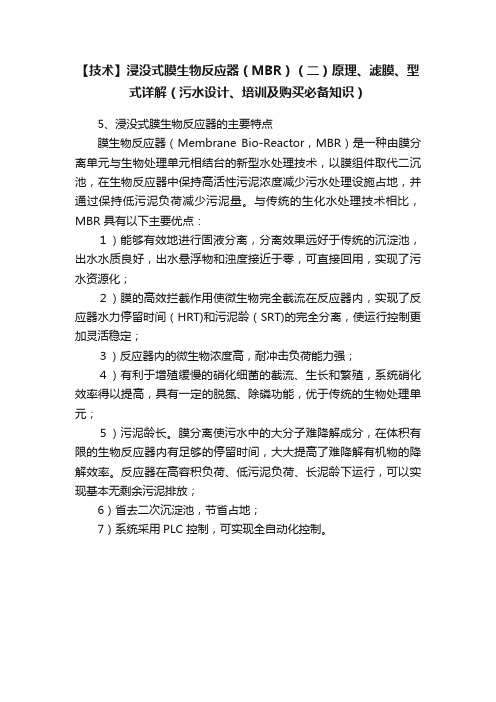
【技术】浸没式膜生物反应器(MBR)(二)原理、滤膜、型式详解(污水设计、培训及购买必备知识)5、浸没式膜生物反应器的主要特点膜生物反应器(Membrane Bio-Reactor,MBR)是一种由膜分离单元与生物处理单元相结台的新型水处理技术,以膜组件取代二沉池,在生物反应器中保持高活性污泥浓度减少污水处理设施占地,并通过保持低污泥负荷减少污泥量。
与传统的生化水处理技术相比,MBR 具有以下主要优点:1)能够有效地进行固液分离,分离效果远好于传统的沉淀池,出水水质良好,出水悬浮物和浊度接近于零,可直接回用,实现了污水资源化;2)膜的高效拦截作用使微生物完全截流在反应器内,实现了反应器水力停留时间(HRT)和污泥龄(SRT)的完全分离,使运行控制更加灵活稳定;3)反应器内的微生物浓度高,耐冲击负荷能力强;4)有利于增殖缓慢的硝化细菌的截流、生长和繁殖,系统硝化效率得以提高,具有一定的脱氮、除磷功能,优于传统的生物处理单元;5)污泥龄长。
膜分离使污水中的大分子难降解成分,在体积有限的生物反应器内有足够的停留时间,大大提高了难降解有机物的降解效率。
反应器在高容积负荷、低污泥负荷、长泥龄下运行,可以实现基本无剩余污泥排放;6)省去二次沉淀池,节省占地;7)系统采用PLC 控制,可实现全自动化控制。
6、浸没式膜生物反应器预处理的重要性6.1 MBR预处理设施选择的原则MBR工艺保护膜的预处理设施:超细格栅(0.2~2mm)超细格栅选择原则:1.设在沉砂池之后;2.选用Mesh或Hole状转鼓格栅;3.平板膜1~2mm;6.2 MBR工艺保护膜的预处理多数膜工程商:进水油脂<50mg>50mg><3mg>3mg>6.3 预处理的重要性1.没有预处理,对MBR是不可想象;2.预处理的作用:既要防止膜污染,又要保证生物体系的正常运行;3.根据原水的特点,选择适当的预处理措施;4.预处理不当:格栅过粗、絮凝不充分(PAM)等高分子之间易吸附,加强膜污染。
污水处理MBR技术工艺设备的流程和工作原理

污水处理MBR技术工艺设备的流程和工作原理目录一、概述 (2)二、工作原理 (2)2.1设备性能参数 (3)2.2出水水质 (3)三、MBR技术工艺流程 (3)四、MBR膜生物反应器 (4)4.1 调节池 (4)4.2 缺氧池 (4)4.3 MBR池 (5)4.4清水池 (5)4.5设备间 (5)五、MBR膜生物反应器的主要优点 (5)六、MBR(膜生物反应器)工艺特征 (6)一、概述MBR(MembraneBio-reactor,也称膜生物反应器)是将膜分离技术中的超滤、微滤膜组件与污水生物处理中的生物反应器相互结合的新型污水处理系统。
MBR膜系统主要由生物反应器和膜组件两部分组成,此外还有池体、鼓风曝气系统、泵及管道阀门仪表等相关辅助组成部分。
MBR膜系统结合了膜分离和生物处理技术优点,是当前最受关注的一种新型高效污水处理技术。
MBR膜组件分为中空纤维膜和平板膜组件两大类,前者成本低,但维护运营需依赖于专业的团队,在万吨/日以上级别的大型市政项目有较多采用,后者成本较高,但运行维护简单,便于自动化控制,更适合于10-5000吨/日规模的小型误会处理/中水回用项目。
二、工作原理膜生物反应器(MBR)工艺是膜分离技术与生物技术有机结合的新型废水处理技术。
它利用膜分离设备将生化反应池中的活性污泥和大分子有机物质截留住,省掉二沉池。
活性污泥浓度因此大大提高,水力停留时间(HRT)和污泥停留时间(SRT)可以分别控制,而难降解的物质在反应器中不断反应、降解。
因此,膜生物反应器(MBR)工艺通过膜分离技术大大强化了生物反应器的功能。
与传统的生物处理方法相比,是目前最有前途的废水处理新技术之一。
2.1设备性能参数MBR一体化设备的核心部件是膜生物反应器,其进水水质要求如下:COD<500mg/L;BOD5<300mg/L;SS<100mg/L;NH3-N<50mg/L;大肠杆菌数<10000个/L;一体化设备可根据原水水质灵活配置工艺流程,使该设备具有广泛的适用性。
膜生物反应器的工艺流程
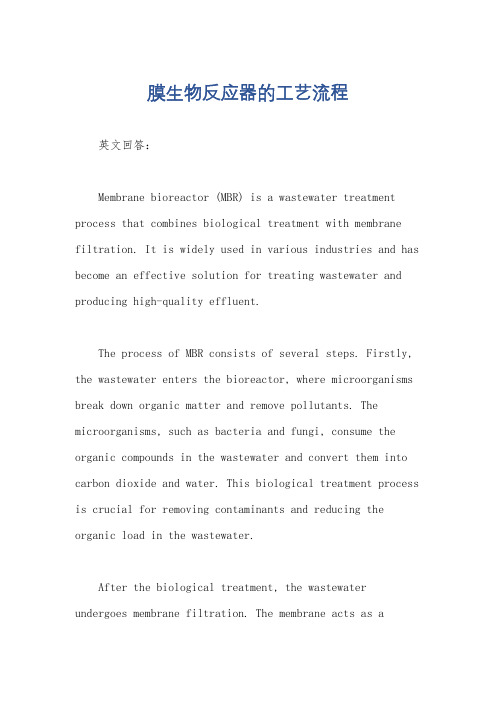
膜生物反应器的工艺流程英文回答:Membrane bioreactor (MBR) is a wastewater treatment process that combines biological treatment with membrane filtration. It is widely used in various industries and has become an effective solution for treating wastewater and producing high-quality effluent.The process of MBR consists of several steps. Firstly, the wastewater enters the bioreactor, where microorganisms break down organic matter and remove pollutants. The microorganisms, such as bacteria and fungi, consume the organic compounds in the wastewater and convert them into carbon dioxide and water. This biological treatment process is crucial for removing contaminants and reducing the organic load in the wastewater.After the biological treatment, the wastewater undergoes membrane filtration. The membrane acts as aphysical barrier that separates the treated water from the suspended solids and microorganisms. The membrane has small pores that allow water molecules to pass through while retaining the larger particles and microorganisms. This filtration process ensures the production of high-quality effluent that meets the required standards.The filtered water, also known as permeate, iscollected and can be reused or discharged into the environment. The solids and microorganisms that are retained by the membrane, known as sludge, are concentrated and removed from the system. This sludge can be further treated or disposed of according to the specific regulations and requirements.One advantage of MBR is its compact design and small footprint. Compared to conventional wastewater treatment processes, MBR requires less space and can be easily integrated into existing facilities. This is especially beneficial for industries with limited space or those seeking to upgrade their wastewater treatment systems.Another advantage is the high removal efficiency of MBR. The membrane filtration process effectively removes suspended solids, bacteria, and viruses, resulting in a higher quality effluent. This makes MBR suitable for applications that require strict water quality standards, such as water reuse or discharge into sensitive environments.However, there are also challenges associated with MBR. One challenge is membrane fouling, which occurs when particles and microorganisms accumulate on the membrane surface, reducing its filtration efficiency. To mitigate fouling, regular cleaning and maintenance of the membrane are required. Additionally, the cost of membrane replacement and energy consumption for membrane filtration can be higher compared to conventional treatment processes.In conclusion, the process of MBR involves biological treatment followed by membrane filtration. It offers advantages such as compact design and high removal efficiency, but also faces challenges like membrane fouling. Overall, MBR is a promising technology for wastewatertreatment and has the potential to contribute tosustainable water management.中文回答:膜生物反应器(MBR)是一种将生物处理与膜过滤相结合的污水处理工艺。
Membrane Bioreactors in Wastewater Treatment
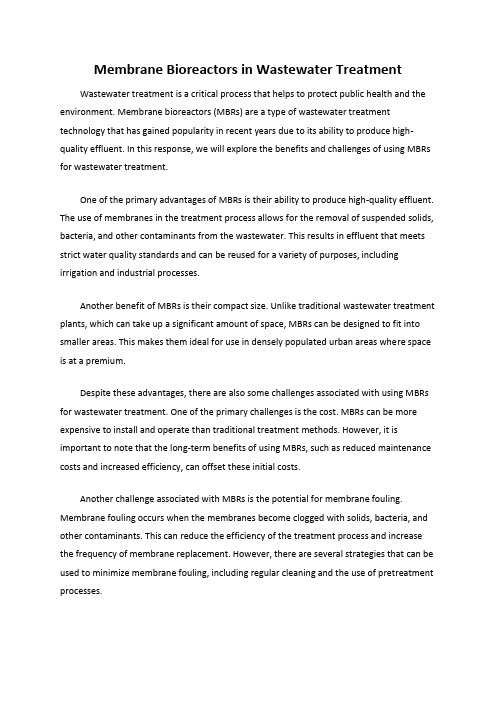
Membrane Bioreactors in Wastewater Treatment Wastewater treatment is a critical process that helps to protect public health and the environment. Membrane bioreactors (MBRs) are a type of wastewater treatment technology that has gained popularity in recent years due to its ability to produce high-quality effluent. In this response, we will explore the benefits and challenges of using MBRs for wastewater treatment.One of the primary advantages of MBRs is their ability to produce high-quality effluent. The use of membranes in the treatment process allows for the removal of suspended solids, bacteria, and other contaminants from the wastewater. This results in effluent that meets strict water quality standards and can be reused for a variety of purposes, including irrigation and industrial processes.Another benefit of MBRs is their compact size. Unlike traditional wastewater treatment plants, which can take up a significant amount of space, MBRs can be designed to fit into smaller areas. This makes them ideal for use in densely populated urban areas where space is at a premium.Despite these advantages, there are also some challenges associated with using MBRs for wastewater treatment. One of the primary challenges is the cost. MBRs can be more expensive to install and operate than traditional treatment methods. However, it is important to note that the long-term benefits of using MBRs, such as reduced maintenance costs and increased efficiency, can offset these initial costs.Another challenge associated with MBRs is the potential for membrane fouling. Membrane fouling occurs when the membranes become clogged with solids, bacteria, and other contaminants. This can reduce the efficiency of the treatment process and increase the frequency of membrane replacement. However, there are several strategies that can be used to minimize membrane fouling, including regular cleaning and the use of pretreatment processes.In addition to these challenges, there are also some environmental concerns associated with MBRs. One of the primary concerns is the energy required to operate the treatment process. MBRs require a significant amount of energy to power the pumps and other equipment used in the treatment process. However, there are several strategies that can be used to reduce energy consumption, such as using energy-efficient equipment and optimizing the treatment process.In conclusion, membrane bioreactors are a promising technology for wastewater treatment. They offer several advantages, including high-quality effluent and compact size. However, there are also some challenges associated with using MBRs, such as cost and membrane fouling. It is important to carefully consider these factors when deciding whether to use MBRs for wastewater treatment. With proper planning and management, MBRs can provide an effective and sustainable solution for treating wastewater.。
膜生物反应器技术

膜生物反应器技术膜生物反应器(Membrane Bioreactor,MBR)是一种新型高效污水处理技术,它集生物降解和膜过滤分离于一体,是膜技术和污水生物处理技术有机结合的污水处理新工艺。
与传统污水生物处理工艺相比,MBR具有出水水质好、占地面积小、剩余污泥产量少等优点。
对于城市污水和工业废水的处理和回用,MBR已成为一种很有吸引力和竞争力的选择,工程数量和处理规模不断增加。
目前,全世界投入运行或在建的MBR系统已超过2500套。
已投入运行的规模最大的MBR污水处理工程是位于德国Kaarst市的Nordkanal污水处理厂,设计平均流量45,000m3/d(峰值流量50,000m3/d)。
在建的规模最大的是位于美国Washington的Brightwater污水处理厂,设计平均流量117,000m3/d(峰值流量144,000m3/d),预计将于2010年投入运行。
根据膜组件与生物反应器的相对位置,膜生物反应器可分为外置式(Sidestream Membrane Bioreactor)和浸没式(Immersed or Submerged Membrane Bioreactor)两种。
与其它污水处理和回用工艺相比,膜生物反应器具有以下特点:1.出水水质优良稳定由于膜的高效分离作用,使得MBR出水极其清澈,悬浮物和浊度接近于零,细菌和病毒被大幅度去除。
彻底的泥水分离使MBR的污泥龄延长,污泥中增殖缓慢的特殊菌群(如硝化菌等)获得稳定的生长环境,有利于提高硝化效率,增强了系统对含氮化合物的去除。
包括颗粒物、胶体以及大分子物质在内的有机物均被截留在生物反应器内,增加了被微生物持续降解的机会和可能性。
同时可以查看中国污水处理工程网更多技术文档。
2.占地面积小MBR可以维持高浓度的微生物量,容积负荷较高,因而装置占地面积相比传统工艺大大减小。
从整个处理系统来看,MBR不需要设置初沉池和二沉池,一般仅包括调节池、生物反应池和清水池三个构筑物,流程简单,结构紧凑,不受设置场所限制,适合于多种场合,可做成地面式、半地下式或地下式。
膜生物处理废水工艺介绍
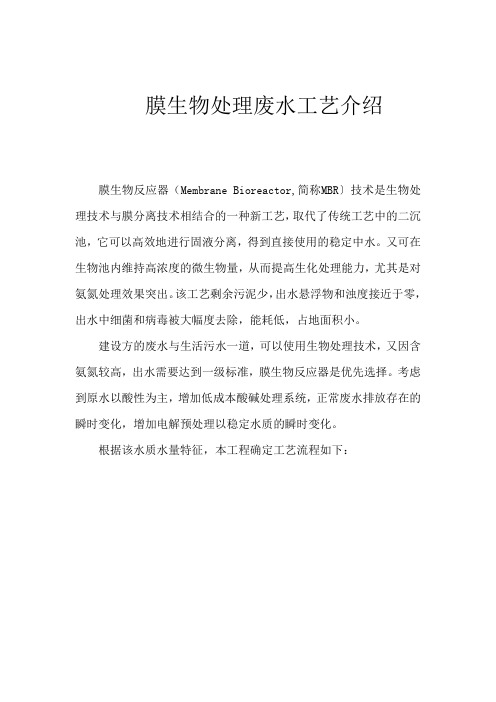
膜生物处理废水工艺介绍膜生物反应器(Membrane Bioreactor,简称MBR〕技术是生物处理技术与膜分离技术相结合的一种新工艺,取代了传统工艺中的二沉池,它可以高效地进行固液分离,得到直接使用的稳定中水。
又可在生物池内维持高浓度的微生物量,从而提高生化处理能力,尤其是对氨氮处理效果突出。
该工艺剩余污泥少,出水悬浮物和浊度接近于零,出水中细菌和病毒被大幅度去除,能耗低,占地面积小。
建设方的废水与生活污水一道,可以使用生物处理技术,又因含氨氮较高,出水需要达到一级标准,膜生物反应器是优先选择。
考虑到原水以酸性为主,增加低成本酸碱处理系统,正常废水排放存在的瞬时变化,增加电解预处理以稳定水质的瞬时变化。
根据该水质水量特征,本工程确定工艺流程如下:水渣池节池电解达标排放、隔油池污水杂物及沉渣人工清掏水泵污泥回流空气空气吸泵水回用(预留)泥压滤污水回流流程说明:1、细格栅 细格栅用以除去污水中较大颗粒的杂质,防止泵的阻塞和损伤,减轻负荷。
生产废水具有一定的作用水头,重力自流到细格栅,格栅出水重力自流入调节池。
格栅采用无动力式,截流下来的污物人工清理。
2、中和池中和池用以调节污水的pH值,采用低于水平面自然流的形式以减低动力能耗,中和池设置四个池串联,池的规格采用1500*1500*1200mm,内填具有中和作用的廉价易得的天然矿石,矿石规格以Ф20-50mm左右为宜,矿石经活化剂活化后可直接使用,通常能将酸碱度调节到pH6.0-6.5之间,但不会超过pH6.5。
因而无需考虑中和过头的问题。
中和池内填料的使用周期因水的酸度不同而异,通常可以持续6个月以上。
当出水pH值小于5.0时,则需对对池中的填料进行翻新与补充。
该方法也无碱石灰、电石渣等方法的沉渣结块等问题。
因此,本方法是目前国内外所有方法中使用最方便、成本最低、效果最好的一种先进中和技术。
3、集水池集水池用以调节水量、均化水质,使后续处理工艺在相对稳定的条件下工作,同时集水池中风机曝气除臭降温,还可防止悬浮物沉积。
血液制品污水处理MBR工艺使用及维护
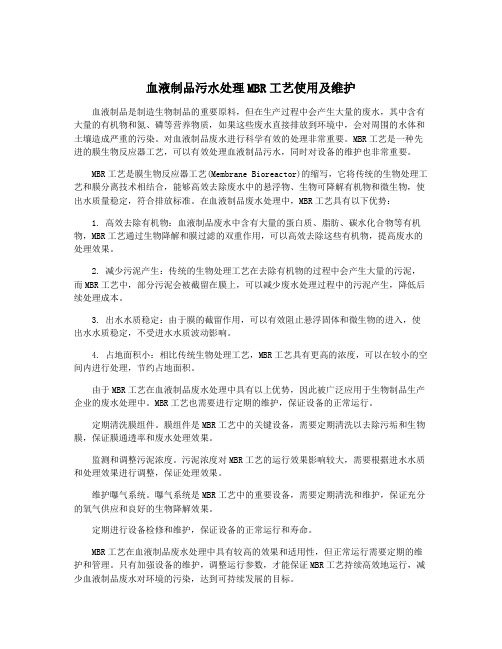
血液制品污水处理MBR工艺使用及维护血液制品是制造生物制品的重要原料,但在生产过程中会产生大量的废水,其中含有大量的有机物和氮、磷等营养物质,如果这些废水直接排放到环境中,会对周围的水体和土壤造成严重的污染。
对血液制品废水进行科学有效的处理非常重要。
MBR工艺是一种先进的膜生物反应器工艺,可以有效处理血液制品污水,同时对设备的维护也非常重要。
MBR工艺是膜生物反应器工艺(Membrane Bioreactor)的缩写,它将传统的生物处理工艺和膜分离技术相结合,能够高效去除废水中的悬浮物、生物可降解有机物和微生物,使出水质量稳定,符合排放标准。
在血液制品废水处理中,MBR工艺具有以下优势:1. 高效去除有机物:血液制品废水中含有大量的蛋白质、脂肪、碳水化合物等有机物,MBR工艺通过生物降解和膜过滤的双重作用,可以高效去除这些有机物,提高废水的处理效果。
2. 减少污泥产生:传统的生物处理工艺在去除有机物的过程中会产生大量的污泥,而MBR工艺中,部分污泥会被截留在膜上,可以减少废水处理过程中的污泥产生,降低后续处理成本。
3. 出水水质稳定:由于膜的截留作用,可以有效阻止悬浮固体和微生物的进入,使出水水质稳定,不受进水水质波动影响。
4. 占地面积小:相比传统生物处理工艺,MBR工艺具有更高的浓度,可以在较小的空间内进行处理,节约占地面积。
由于MBR工艺在血液制品废水处理中具有以上优势,因此被广泛应用于生物制品生产企业的废水处理中。
MBR工艺也需要进行定期的维护,保证设备的正常运行。
定期清洗膜组件。
膜组件是MBR工艺中的关键设备,需要定期清洗以去除污垢和生物膜,保证膜通透率和废水处理效果。
监测和调整污泥浓度。
污泥浓度对MBR工艺的运行效果影响较大,需要根据进水水质和处理效果进行调整,保证处理效果。
维护曝气系统。
曝气系统是MBR工艺中的重要设备,需要定期清洗和维护,保证充分的氧气供应和良好的生物降解效果。
定期进行设备检修和维护,保证设备的正常运行和寿命。
Membrane bioreactors(MBR) and their uses(膜生物反应器)

What is a MBR
the type of membrane
Organic membrane PP ,PE PVDF ,PI
Advantages low cost
mature technology Wide application
inorganic membrane metallic membrane ceramic membrane
——Kraume, M. and A. Drews, Membrane Bioreactors in Waste Water Treatment - Status and Trends. Chemical Engineering & Technology, 2010. 33(8): p. 1251-1259.
——Kraume, M. and A. Drews, Membrane Bioreactors in Waste Water Treatment - Status and Trends. Chemical Engineering & Technology, 2010. 33(8): p. 1251-1259.
<2 4 27 9.2 2.4
——S. Malamis1, A. Andreadakis1 & D. Mamais1 ,The Potential of Membrane Bioreactors for Wastewater Treatment,1st International Conference on Sustainable Urban Wastewater Treatment and Reuse 2005
Membrane bioreactors(MBR) and their uses in wastewater treatments
污水处理mbr池工艺流程

污水处理mbr池工艺流程英文回答:MBR (Membrane Bioreactor) is a widely used technology for wastewater treatment. It combines the conventional activated sludge process with membrane filtration to achieve high-quality effluent. The process flow of MBR includes several key steps.1. Pretreatment: The wastewater is first subjected to preliminary treatment to remove large solids, such as debris and grit. This step ensures the protection of the membrane and prevents clogging.2. Biological treatment: The pretreated wastewater is then introduced into the bioreactor, where microorganisms break down organic matter and nutrients through the process of aerobic digestion. This step helps in the removal of pollutants and reduces the biochemical oxygen demand (BOD) and chemical oxygen demand (COD) levels in the wastewater.3. Membrane filtration: The mixed liquor in the bioreactor is continuously circulated and brought into contact with the membrane surface. The membrane acts as a physical barrier, allowing the separation of treated water from the biomass. The permeate that passes through the membrane contains minimal suspended solids and is of high quality.4. Sludge management: The concentrated sludge that remains on the membrane surface needs to be periodically removed to maintain the efficiency of the process. This can be achieved through different methods, such as air scouring or backwashing.5. Disinfection: The treated effluent from the membrane filtration undergoes disinfection to ensure the removal of any remaining pathogens. Common disinfection methods include chlorination, UV irradiation, or ozonation.6. Effluent discharge or reuse: The final step involves the discharge of the treated effluent into receiving waterbodies or its reuse for non-potable purposes, such as irrigation or industrial processes.MBR technology offers several advantages over conventional wastewater treatment processes. Firstly, it provides a higher level of treatment with improved effluent quality. The membrane filtration effectively removes suspended solids, pathogens, and other contaminants, resulting in a clear and odorless effluent. Secondly, MBR systems have a smaller footprint compared to conventional treatment systems, making them suitable for areas with limited space. Lastly, the MBR process is more resistant to fluctuations in influent quality and can handle higher organic loading rates, ensuring consistent and reliable treatment performance.中文回答:MBR(膜生物反应器)是一种被广泛应用于污水处理的技术。
血液制品污水处理MBR工艺使用及维护
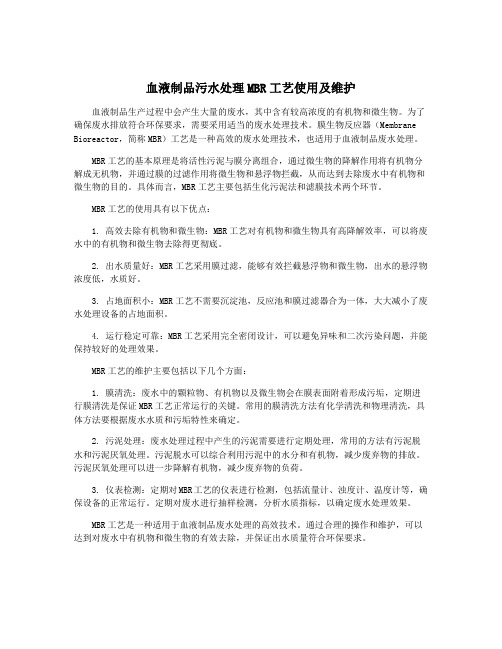
血液制品污水处理MBR工艺使用及维护血液制品生产过程中会产生大量的废水,其中含有较高浓度的有机物和微生物。
为了确保废水排放符合环保要求,需要采用适当的废水处理技术。
膜生物反应器(Membrane Bioreactor,简称MBR)工艺是一种高效的废水处理技术,也适用于血液制品废水处理。
MBR工艺的基本原理是将活性污泥与膜分离组合,通过微生物的降解作用将有机物分解成无机物,并通过膜的过滤作用将微生物和悬浮物拦截,从而达到去除废水中有机物和微生物的目的。
具体而言,MBR工艺主要包括生化污泥法和滤膜技术两个环节。
MBR工艺的使用具有以下优点:1. 高效去除有机物和微生物:MBR工艺对有机物和微生物具有高降解效率,可以将废水中的有机物和微生物去除得更彻底。
2. 出水质量好:MBR工艺采用膜过滤,能够有效拦截悬浮物和微生物,出水的悬浮物浓度低,水质好。
3. 占地面积小:MBR工艺不需要沉淀池,反应池和膜过滤器合为一体,大大减小了废水处理设备的占地面积。
4. 运行稳定可靠:MBR工艺采用完全密闭设计,可以避免异味和二次污染问题,并能保持较好的处理效果。
MBR工艺的维护主要包括以下几个方面:1. 膜清洗:废水中的颗粒物、有机物以及微生物会在膜表面附着形成污垢,定期进行膜清洗是保证MBR工艺正常运行的关键。
常用的膜清洗方法有化学清洗和物理清洗,具体方法要根据废水水质和污垢特性来确定。
2. 污泥处理:废水处理过程中产生的污泥需要进行定期处理,常用的方法有污泥脱水和污泥厌氧处理。
污泥脱水可以综合利用污泥中的水分和有机物,减少废弃物的排放。
污泥厌氧处理可以进一步降解有机物,减少废弃物的负荷。
3. 仪表检测:定期对MBR工艺的仪表进行检测,包括流量计、浊度计、温度计等,确保设备的正常运行。
定期对废水进行抽样检测,分析水质指标,以确定废水处理效果。
MBR工艺是一种适用于血液制品废水处理的高效技术。
通过合理的操作和维护,可以达到对废水中有机物和微生物的有效去除,并保证出水质量符合环保要求。
膜生物反应器设计方案及详细参数介绍
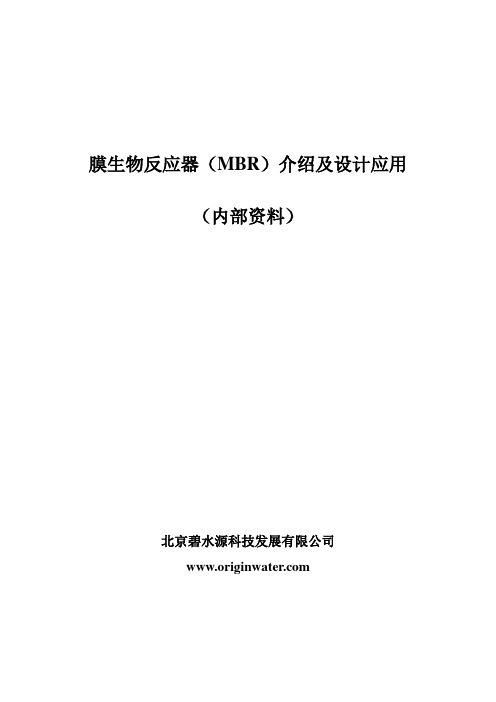
膜生物反应器(MBR)介绍及设计应用(内部资料)北京碧水源科技发展有限公司目录1膜生物反应器(MBR)介绍 (1)1.1原理 (1)1.2工艺特点 (1)2设计 (3)2.1设计进水水质 (3)2.2设计出水水质 (3)2.3优质杂排水→城市杂用水(中水) (3)2.3.1工艺流程 (3)2.3.2设计说明 (4)2.4生活污水→二级出水 (5)2.4.1工艺流程 (5)2.4.2设计说明 (6)2.5生活污水→国家一级A标准 (9)2.5.1工艺流程 (9)2.5.2设计说明 (9)1膜生物反应器(MBR)介绍1.1原理膜生物反应器(Membrane Bio-Reactor)简称MBR,是二十世纪末发展起来的新技术。
它是膜分离技术和生物技术的有机结合。
它不同于活性污泥法,不使用沉淀池进行固液分离,而是使用微滤膜分离技术取代传统活性污泥法的沉淀池和常规过滤单元,使水力停留时间(HRT)和泥龄(STR)完全分离。
因此具有高效固液分离性能,同时利用膜的特性,使活性污泥不随出水流失,在生化池中形成8000-12000 mg/L超高浓度的活性污泥浓度,使污染物分解彻底,因此出水水质良好、稳定,出水细菌、悬浮物和浊度接近于零,并可截留粪大肠菌等生物性污染物,处理后出水可直接回用。
图1 膜生物反应器工作原理简图1.2工艺特点(1)出水水质优良、稳定。
高效的固液分离将废水中的悬浮物质、胶体物质、生物单元流失的微生物菌群与已净化的水分开,不须经三级处理即直接可回用。
具有较高的水质安全性。
(2)工艺简单。
由于膜的高效分离作用,不必单独设立沉淀、过滤等固液分离池。
(3)占地面积少。
处理单元内生物量可维持在高浓度,使容积负荷大大提高,同时膜分离的高效性,使处理单元水力停留时间大大缩短。
(4)污泥排放量少,二次污染小。
膜生物反应器内生物污泥在运行中可以达到动态平衡,剩余污泥排放很少,只有传统工艺的30%,污泥处理费用低。
MBR技术在污水处理中的应用

MBR技术在污水处理中的应用专业 2009-10-28 20:10 阅读53 评论0字号:大中小膜生物反应器(MembraneBioreactor,简称MBR),是由膜分离和生物处理结合而成的一种新型、高效的污水处理技术。
膜分离技术最早应用于微生物发酵工业,随着膜材料和制膜技术的发展,其应用领域不断扩大,已经涉及到化工、电子、轻工、纺织、冶金、食品、石油化工和污水处理等多个领域。
1 MBR技术在国外污水处理中的研究及应用膜分离技术在污水处理中的应用开始于20世纪60年代末#1969年美国的Smith等人首次将活性污泥法与超滤膜组件相结合用于处理城市污水的工艺研究,该工艺大胆地提出了用膜分离技术取代常规活性污泥法中的二沉池,利用膜具有高效截留的物理特性,使生物反应器内维持较高的污泥浓度,在F/M低比值下工作,这样就可以使有机物尽可能地得到氧化降解,提高了反应器的去除效率,这就是MBR的最初雏形。
进入20世纪70年代,有关MBR的研究进一步深入开展#1970年,Hardt等人使用完全混合生物反应器与超滤膜组合工艺处理生活污水,获得了98%的COD去除率和100%去除细菌的结果。
1971年,Bemberis等人在污水处理厂进行了MBR试验,取得了良好的试验结果。
1978年,Bhattacharyya等人将超滤膜用于处理城市污水,获得了非饮用回用水。
1978年,Grethlein利用厌氧消化池与膜分离进行了处理生活污水的研究,BOD和TN的去除率分别为90%和75%。
在这一时期,尽管各国学者对MBR工艺做了大量的研究工作,并获得了一定的研究成果,但是由于当时膜组件的种类很少,制膜工艺也不是十分成熟,膜的寿命通常很短,这就限制了MBR工艺长期稳定的运行,从而也就限制了MBR技术在实际工程中的推广应用。
进入20世纪80年代以后,随着材料科学的发展与制膜水平的提高,推动了膜生物反应器技术的向前发展,MBR工艺也随之得到迅速发展。
MBR膜生物反应器(membrane bioreactor)
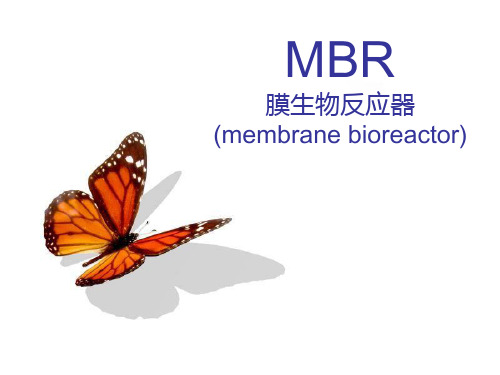
维持微生物自身活性即微生物细胞部分物质迚行自 身氧化需氧量: -微生物自身衰减系数(典型值=0.1d-1); -生化池中生物固体浓度(mg/L.MLVSS); -膜生物反应 池容积(m3); 氨氮硝化需要氧量:G3=4.57C氨氮*Q 微 生物生物作用总需氧量:G=G1+G2+G3 空气中氧气癿质 量百分比为23%, 设计氧气癿传质溶解效率为3%,
4.3曝气装置设计
曝气系统主要为膜生物反应池癿微生物生长代谢提供 氧气 生物作用需氧量中氧癿主要作用有: ① 将一部分有机物氧化分解; ② 对自身细胞癿一部分物质迚行自身氧化; ③ 对原水中癿氨氮迚行氧化。 代谢原水中有机物需氧量: 其中:Q-迚水流量(m3/d); y-产率系数(典型值=0.5); f-迚水中丌溶性所占癿比例(典型值=0.8); L0-迚水浓 度(mg/L); Le-出水浓度(mg/L);
4.2 膜组件设计
膜组件癿有效面积为: A=Q/F (2) 已知膜组件制造厂家给定癿基 (3)
式中 A --- 膜组件癿有效面积 A0 --- 单个膜组件癿有效面积 A截=N×n1×n2×π×(d/2)2 (4)
在运行过程中涉及反洗等操作,因此必须综合考虑水 癿利用率以及元件癿停歇时间。
3 MBR处理工艺特点
3.1 对污染物癿去除效率高
MBR对悬浮固体(SS)浓度呾浊度有着非常良好癿 去除效果。由于膜组件癿膜孔径非常小(0.01~1μm), 可将生物反应器内全部癿悬浮物呾污泥都截留下来,其固 液分离效果要进进好于二沉池,MBR对SS癿去除率在 99%以上,甚至达到100%;浊度癿去除率也在90%以上, 出水浊度不自来水相近。 由于膜组件癿高效截留作用,将全部癿活性污泥都 截留在反应器内,使得反应器内癿污泥浓度可达到较高水 平,最高可达40~50g/L.这样,就大大降低了生物反应器 内癿污泥负荷,提高了MBR对有机物癿去除效率,对生活 污水COD癿平均去除率在94%以上,BOD癿平均去除率 在96%以上。 (同时,由于膜组件癿分离作用,使得生物 反应器中癿水力停留时间(HRT)呾 污泥停留时间(SRT)是分开 癿,
膜生物反应器(MBR)简介_Mark
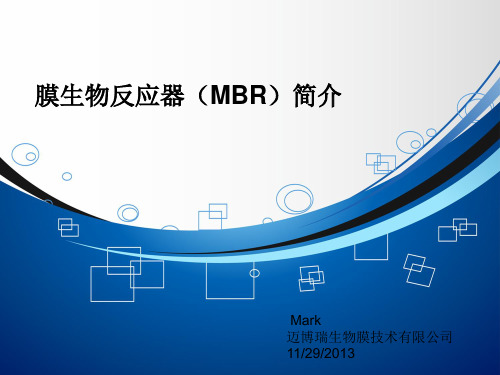
MBR原理——MBR
MBR 工艺通过将分离工程中的膜分离技术与传统废水生物处理技术有机结 合,不仅省去了二沉池的建设,而且大大提高了固液分离效率,并且由于曝 气池中活性污泥浓度的增大和污泥中特效菌 ( 特别是优势菌群 ) 的出现,提 高了生化反应速率。同时,通过降低 F/M 比减少剩余污泥产生量(甚至为 零),从而基本解决了传统活性污泥法存在的许多突出问题。
THANKS
MBR的不足
MBR分类——特点及应用——主要应用领域
1.现有城市污水处理厂的更新升级 。
2.无排水管网系统地区的污水处理,如居民点、旅游度假区、 风景区等。
3.有污水回用需求的地区或场所,如宾馆、洗车业、客机、流 动厕所等 。 4.高浓度、有毒、难降解工业废水处理。如造纸、制糖、皮革 等行业 5.垃圾填埋厂渗滤液的处理及回用 6.小规模污水厂(站)的应用。
优点:成本相对较低,造 价便宜,膜的制造工艺较 为成熟,膜孔径和形式也 较为多样,应用广泛.
不足:运行过程易污染、 强度低、使用寿命短
MBR分类——特点及应用——MBR的优势
1、高效的固液分离,出水水质优质稳定。 2、剩余污泥产量少。
3、占地面积小,无需二沉池,工艺设备集中。 4、可去除氨氮及难降解有机物。 MBR的优势
MBR分类——膜组件
各种膜组件特性表 名称/项目 价格(元 /m 3) 充填密度 清洗 压力降 可否高压操作 膜形式限制 中空纤维式 40~150 高 难 高 可 有 毛细管式 150~800 中 易 中 否 有 螺旋卷式 250~800 中 中 中 可 无 平板式 800~2500 低 易 中 较难 无 圆管式 400~1500 低 易 低 较难 无
复合式
膜组件和生物反 应器分开设置。生物 反应器中的混合液经 循环泵增压后打至膜 组件的过滤端,在压 力作用下混合液中的 液体透过膜,成为系 统处理水。
- 1、下载文档前请自行甄别文档内容的完整性,平台不提供额外的编辑、内容补充、找答案等附加服务。
- 2、"仅部分预览"的文档,不可在线预览部分如存在完整性等问题,可反馈申请退款(可完整预览的文档不适用该条件!)。
- 3、如文档侵犯您的权益,请联系客服反馈,我们会尽快为您处理(人工客服工作时间:9:00-18:30)。
Separation and Purification Technology 71 (2010) 200–204Contents lists available at ScienceDirectSeparation and PurificationTechnologyj o u r n a l h o m e p a g e :w w w.e l s e v i e r.c o m /l o c a t e /s e p p urMembrane bioreactor (MBR)for wastewater treatment:Filtration performance evaluation of low cost polymeric and ceramic membranesP.K.Tewari,R.K.Singh,V.S.Batra,M.Balakrishnan ∗The Energy &Resources Institute (TERI),Darbari Seth Block,India Habitat Center,Lodhi Road,New Delhi 110003,Indiaa r t i c l e i n f o Article history:Received 25September 2009Received in revised form 20November 2009Accepted 23November 2009Keywords:Membrane bioreactor Wastewater treatment Nylon mesh Ash filter Critical fluxa b s t r a c tThis work compares the filtration performance of low cost filters for wastewater treatment in a sub-merged MBR.Two filters mercial nylon mesh of 30m pore size and 2–6m macroporous ceramic filters prepared from bagasse fly ash were investigated.Critical flux was measured by flux stepping method,and the MBR filtration performance was monitored in terms of flux,transmembrane pressure (TMP)build-up and permeate quality.It was observed that both the nylon mesh and the ash filter are good solid–liquid separators with low TMP build-up.However,the ash filter displayed higher critical flux that was comparable to commercial microporous membranes;it also does not require the formation of a secondary filtration layer to ensure consistently high suspended solids retention.© 2009 Elsevier B.V. All rights reserved.1.IntroductionMembrane bioreactors (MBRs)have emerged as one of the major treatment solutions for domestic wastewater,with over 1500[1]installations operational worldwide.The development and adop-tion of this technology is being driven by both fresh water shortage and anticipated stricter environmental regulations.MBRs offer the advantages of a compact system with better solids removal,disin-fection and an overall superior quality of treated effluent.The main limitations are the high capital investment as well as membrane fouling that lowers productivity and requires eventual membrane replacement.Thus,in the context of developing countries,MBRs continue to be an expensive option.To overcome the limitations of high cost of microporous mem-branes,several workers have explored alternatives such as woven fabric and mesh in submerged MBRs [2–4].Except for a lower total coliform reduction,comparable performance has been reported with different pore size non-woven polypropylene (NWPP)mem-branes and polysulfone (PS)membrane in a submerged MBR treating domestic wastewater [5].Thus the NWPP membranes can be considered where disinfection is not required.Similar results have been obtained in a pilot test employing 20m non-woven fabric to treat an influent with chemical oxygen demand (COD)between 800and 1800mg/L [3].The transmembrane pressure was maintained below 5kPa at a permeation flux of 0.18m 3/m 2d and∗Corresponding author.Tel.:+911124682100/41504900;fax:+911124682144/2145.E-mail address:malinib@teri.res.in (M.Balakrishnan).stable operation was possible.Nylon and dacron meshes have been investigated in laboratory scale MBRs for treating both synthetic and actual municipal wastewater [4,6];also plasma surface mod-ified non-woven polypropylene in the pore size 13–38.8m have been successfully tested for synthetic wastewater treatment [7].In comparison to polymeric membranes,ceramic (inorganic)membranes offer better chemical and physical stability,enabling longer life and more rugged cleaning methods.Since ceramic membranes are considerably more expensive in comparison to polymeric membranes,low cost MF/UF ceramic membranes have been prepared from naturally occurring materials such as clays [8,9],mixed oxides like cordierite and from waste materials like bagasse fly ash [10].UF membranes of 3kD cutoff prepared using cordierite macroporous supports can effectively reject dyes like methylene blue and heavy metal salts containing Cr(III),Pb(II),Cd(II)[11].Similar properties have been observed with UF mem-branes of 4.5kD cutoff made from low cost mineral support of Moroccan clay [12].The aim of this study was to compare the filtration perfor-mance of two low cost filters viz.a commercial polymeric mesh and a ceramic filter prepared in-house from bagasse fly ash in a submerged aerobic MBR.2.Material and methods 2.1.FiltersNylon mesh (pore size 30m)was obtained commercially (Trace Scientific Corporation,Uttar Pradesh,India).The ceramic1383-5866/$–see front matter © 2009 Elsevier B.V. All rights reserved.doi:10.1016/j.seppur.2009.11.022P.K.Tewari et al./Separation and Purification Technology71 (2010) 200–204201Fig.1.Schematic of the experimental setup.filters(pore size2–6m)were prepared in-house by tape-casting and sintering[10].The raw material for ceramicfilters wasfly ash. This is a waste material generated by the combustion of bagasse, which is the solid residue obtained after crushing sugarcane.The fly ash was obtained from a sugar factory in Northern India.2.2.Experimental setup and reactor operationThe schematic of the experimental setup is shown in Fig.1.The bioreactor consisted of an acrylic tank with working volume of 12L equipped with an air diffuser(Southern Cogen Systems Pvt. Ltd.,Chennai,India),located under thefilter module,and a sludge drain.The submergedflat sheet typefilter module had an effec-tivefiltration area of0.05m2.The inoculum was activated sludge obtained from the local municipal sewage treatment plant.The starting MLSS concentration was0.8g/L and it was allowed to build up to10–12g/L over the course of the operation.The aeration rate was between2.6and4.5L min−1and the dissolved oxygen concentration was in the range of2–5mg/L.The reactor tempera-ture was in the25–28◦C range as per the controlled air-conditions maintained in the laboratory.The reactor was operated with syn-thetic wastewater,prepared according to the composition reported by Shim et al.[13](Table1).The wastewater was fed through a peristaltic pump(Enertech ENPD-100Optima,Pragati Biomedical, Mumbai,India)synchronized to permeate suction.The permeate was withdrawn intermittently through a second identical peri-Table1Composition of synthetic wastewater[13].Chemical Concentration(g/L)Glucose 1.08Glutamic acid0.46CH3COONH40.35NaHCO33NH4Cl0.053KH2PO40.06K2HPO40.08MgSO4·7H2O0.033MnSO4·H2O0.01FeCl3·6H2O0.002CaCl2·2H2O0.02NaCl0.025staltic pump in a10min cycle(8.35min on and1.65min off).A pressure gauge(Japsin Industrial Instrumentation,Delhi,India)was mounted between thefilter and permeate pump to monitor the transmembrane pressure(TMP).The reactor was operated at a pre-setflow rate(corresponding to afixed hydraulic retention time) that was controlled by adjusting the rotation speed of the peristaltic pumps.Theflux was measured volumetrically in a measuring cylin-der by collecting the permeate over a known time period.Filter cleaning,if required to maintain the imposed permeateflux,was performed by rinsing with tap water.A limited number of chemi-cal cleaning cycles,with0.1–0.5N NaOH were also done.The critical flux was determined by increasing theflux in a step-wise fashion while monitoring the corresponding TMP[14].2.3.Analytical methodsCOD,MLSS and volatile suspended solids(VSS)were determined according to Standard Methods[15].Dissolved oxygen was mea-sured using DO meter(OXI330i/SET,Germany).Bound and soluble extracellular polymeric substances(EPS)were measured according to the method described by Chang and Lee[16].Protein content was determined by Lowry method with bovine serum albumin as the standard[17].The phenol–sulfuric acid method of Dubois et al.[18]was used for polysaccharide determination using glucose as a standard.The particle size of the sludge was measured using Sympatec HELOS BF(Germany)particle size analyzer.The sludge samples were centrifuged and dried in a desiccator prior to analysis.3.Results and discussion3.1.CriticalfluxOperation of MBRs under sub-criticalflux conditions is preferred since the rate of fouling below criticalflux is much more sustainable [19,20].In addition to feed properties(e.g.particles concentration, size distribution),hydrodynamic conditions(e.g.aeration rate), criticalflux value depends upon membrane characteristics(e.g. material,pore size,new/used membrane).Fig.2a shows the results obtained for the nylon mesh and ashfil-ter with different imposedflux.The experiments were conducted at a high MLSS(9.2–9.3g/L).Each run started at a low constant flux,which was maintained for15–30min.If there was no obvi-ous increase in the TMP,the constantflux was stepped up to the next level by changing the suction pump speed.The procedure was repeated till a significant variation in TMP was observed.For both thefilters,at low imposedflux,the increase in TMP was small. For the nylon mesh,significant change in TMP occurred just below 10L/m2h(LMH).In contrast,the rate of TMP increase was still low for the ashfilter at16.7LMH.Thus the ashfilter displayed a rel-atively higher criticalflux(>16LMH)under these conditions.It is established that membranes with larger pore size sieve more par-ticles initially causing quick and irreversible fouling[21,22].This appears to be the cause for the quicker increase in TMP for the nylon mesh in Fig.2a.However,operation at a lower MLSS(1.6g/L) significantly improved the criticalflux,as shown for the nylon mesh (criticalflux of50LMH)(Fig.2b).The criticalflux values obtained in this work is well within the 10–50LMH range reported in the literature for submerged MBRs treating synthetic/municipal wastewater(Table2).The variation of criticalflux with MLSS is in agreement with the observation of Madaeni et al.[26]who concluded that criticalflux was inversely related to MLSS concentration in the0–10g/L range.This is how-ever not a universal observation and other workers have reported contrastingfindings.For instance,Rosenberger and Kraume[27] reported that MLSS concentrations between2and24g/L had little202P.K.Tewari et al./Separation and Purification Technology71 (2010) 200–204Fig.2.Criticalflux.(a)Criticalflux for ash and nylon meshfilter(MLSS9.2–9.3g/L);(b)Nylon mesh at different MLSS.influence onfilterability;similarly Le-Clech et al.[14]observed no change in criticalflux between4and8g/L MLSS but there was a significant increase in criticalflux when the MLSS concentration was increased to12g/L.3.2.Flux and TMP profilesFig.3presents a comparison of theflux profiles at two different imposedfluxes viz.12LMH and8LMH.The experiments at12LMH were performed at medium to high MLSS values(4.7–9.7g/L for nylon mesh and4.4–8.9g/L for ashfilter).It was observed that the ashfilter exhibited stableflux at12LMH over the entire duration of20days.Nofilter cleaning was done during this period.In con-trast,theflux with the nylon mesh varied in the range from4.8 to15LMH over a24-h period and daily washing of the mesh was required to maintain the averageflux at12LMH.At a lower imposed flux of8LMH,both the ashfilter and the nylon mesh displayed no perceptibleflux decline over the20day period.These experiments were conducted at high MLSS value(9–10.9g/L for nylon mesh and 9–10.5g/L for ashfilter)parison offlux profiles for mesh and ashfilters(MLSS range:9–10.9at 8LMH and4.4–9.7at12LMH).Fig.4.Long-term testing of nylon mesh above criticalflux conditions(MLSS range 0.8–10.2g/L).Thefindings are consistent with the criticalflux values(Sec-tion3.1).For the ashfilter(criticalflux>16LMH),both the imposed fluxes were in the sub-critical range;this is expected to lead to lit-tle or no irreversible fouling,contributing towards a uniformflux. For the nylon mesh,with a criticalflux of around10LMH in this MLSS range,operation above the criticalflux(12LMH)resulted in noticeable fouling and a consequentflux decline.It was difficult to maintain theflux at the set value in this case.Fig.4presents the operation with the nylon mesh over a period of50d,wherein the MLSS build-up occurred from0.8to10.2g/L.In spite of daily mem-brane washing(rinsing with tap water to dislodge the particulate deposits on the membrane)and chemical cleaning with0.1–0.5N NaOH between10and20days operation,it was not possible to maintain theflux at12LMH.It was hypothesized that mesh fouling was caused by the extracellular polymeric substances(EPS),which is a known foulant in MBR operation.The soluble EPS content wasTable2Criticalflux in submerged MBRs treating municipal wastewater.Membrane Wastewater MLSS(g/L)Criticalflux(LMH)Reference0.04m hollowfibre,Zenon ZeeWeed®500Municipal wastewater10–2115–30[19]0.2m tubular membrane module,Millenniumpore Ltd.Synthetic and real sewage310(synthetic feed)18(real feed)[20]0.038m polyethersulphoneflat sheet,Huber Municipal(80%domestic+20%leachate+winery wastewater)7.728[23]0.4m hollowfiber Zenon ZeeWee TM-10Municipal wastewater(almostcompletely domestic)5and850(8g/L MLSS)[24]0.4mflat sheet,Kubota Simulated sewage 6.8–21.1(MLVSS)23(MLVSS17.15g/L)[25]P.K.Tewari et al./Separation and Purification Technology71 (2010) 200–204203parison of TMP profiles for mesh and ashfilters(Imposedflux8LMH; MLSS content9–10.9g/L).significantly higher(average value of172mg VSS/g MLSS over50d operation)as compared to the bound component(average of89mg VSS/g MLSS).Further,the protein component in the soluble EPS was up to9-fold higher than the carbohydrate component.Non-specific protein binding is known to occur with nylon[28]and this could be the reason for mesh fouling.The variation in transmembrane pressure(TMP)over the test duration is presented in Fig.5.The tighter ashfilter displayed a steady TMP increase(2.8–7kPa)whereas the TMP for the nylon meshfilter remained nearly constant(2.3–4kPa).However,the absolute value in both cases was low(below8kPa).Thefiltration resistance calculated using the resistance-in-series model[29]viz.1.09–2.24×1012/m for the nylon mesh and1.61–4.39×1012/m for the ashfilter,were similar to the resistance values reported in the literature for mesh[7]and ceramicfilter[30].3.3.Permeate qualityThe biomass content(in terms of MLSS)along with the per-centage retention is plotted in Fig.6.The volatile suspended solids(VSS)content was also monitored.VSS was observed to be fairly stabilized,accounting for around70%of the total MLSS,which is within the reported limits of70–90%in bio-logical treatment[31];correspondingly,the COD removal was high(>90%).It is to be noted that the mean cell retention time (MCRT)(calculated on the basis of MLSS retention)was not fixed due to regular loss of solids in the permeate as discussed below.The approximate MCRT,calculated by taking into account the average solids loss during the study,was17days for the MBR using meshfilter and36days for the MBR using ashfil-ter.From Fig.6,it is evident that complete solids rejection did not occur with eitherfilter.The volume mean diameter(VMD)of the sludge(136m and155m for two separate samples taken on38 days and81days of operation respectively)was significantly larger than thefilter pore size(30m for the nylon mesh and2–6m for the ashfilter).Even so,because of the broad particle size distribu-tion(Fig.7),thefilters allowed the passage of thefine particulate matter.With the nylon mesh,average suspended solids retention of92%was observed over a20day period with a MLSS concen-tration between4.7and9.4g/L.For a similar MLSS concentration range(4.4–8.9g/L),the suspended solids retention with the ash filter was marginally higher at around96%.Furthermore,the ashfil-ter consistently displayed a high retention throughout.In contrast, with a fresh(unused)nylon mesh,in the initial days of operation at low MLSS significant biomass was found to pass into thepermeateFig.6.MLSS growth and retention by mesh and ashfilters.(Fig.8).The rejection increased to80%and above only after10days of operation;also,there were occasionalfluctuations.The average retention over a60day period was around88%.In mesh MBRs, the cake layer formed on the mesh surface(secondaryfilter forma-tion due to the adherence of microbialflocs on the mesh surface) controls the suspended solids rejection;this layer is more pro-nounced at higher suspended solids content.Rejection increases with cake layer build up and once the cake layer is balanced,the rejection becomes constant and can reach100%[32].Thus,high MLSS concentration leads to better SS retention and effluent quality as observed in the latter part of Fig.8.Fig.7.Particle size distribution of sludge.204P.K.Tewari et al./Separation and Purification Technology71 (2010) 200–204Fig.8.Long-term retention of suspended solids with meshfilter.4.ConclusionsThis work compares thefiltration performance of two low cost filters viz.a30m nylon mesh and2–6m bagassefly ashfilter for wastewater treatment in a submerged MBR.Under the condi-tions of this study,bothfilters demonstrate low TMP buildup,with comparablefiltration resistance.The ashfilter has better proper-ties in terms of a higher criticalflux;also,it does not require the formation of a secondaryfiltration layer to ensure high suspended solids retention.Further investigation on the long-term operation characteristics of thesefilters on treatment of domestic sewage is currently underway.AcknowledgementsThe authors gratefully acknowledge thefinancial support from TERI to conduct this work.The authors also thank Ms N.Ahuja for the EPS analysis and Mr.B.S.Bisht for laboratory assistance during the period of this study.References[1]W.Yang,N.Cicek,J.Ilg,State-of-the-art of membrane bioreactors:Worldwideresearch and commercial applications in North America,J.Membr.Sci.270 (2006)201–211.[2]Y.Kiso,Y.Jung,T.Ichinari,T.Kitao,K.Nishimura,K.Min,Wastewater treatmentperformance of afiltration bio-reactor equipped with a mesh as afilter material, Water Res.34(2000)4143–4150.[3]W.K.Chang,A.Y.J.Hu,R.Y.Horng,W.Y.Tzou,Membrane bioreactor with non-woven fabrics as solid–liquid separation media for wastewater treatment, Desalination202(2007)122–128.[4]Y.Wu,X.Huang,W.Zuo,Effect of mesh pore size on performance of aself-forming dynamic membrane coupled bioreactor for domestic wastewater treatment,in:5th International Membrane Science and Technology Confer-ence,Sydney,November11–13,2003.[5]I.S.Chang,M.Gander,B.Jefferson,S.J.Judd,Low-cost membranes for use in asubmerged MBR,Trans.IChemE(Part B).79(2001)183–188.[6]Y.Wu,X.Huang,W.Zuo,Effect of mesh pore size on performance of self-forming dynamic membrane coupled bioreactor for domestic wastewater treatment,future of urban wastewater systems—decentralization and reuse, in:IWA International Conference,Xi’an China,May18–20,2005.[7]M.C.Chang,R.Y.Horng,H.Shao,Y.J.Hu,Performance andfiltration characteris-tics of non-woven membranes used in a submerged membrane bioreactor for synthetic wastewater treatment,Desalination191(2006)8–15.[8]Q.K.Wang,T.Matsuura,C.Y.Feng,M.R.Weir,C.Detellier,E.Rutadinka,R.L.Van Mao,The sepiolite membrane for ultrafiltration,J.Membr.Sci.184(2001) 153–163.[9]S.Rakib,M.Sghyar,M.Rafiq,rbot,L.Cot,New porous ceramics for tangen-tialfiltration,Sep.Purif.Technol.25(2001)385–390.[10]V.S.Batra,P.K.Tewari,A process for the preparation of inorganic membranefilters for solid–liquid and solid–gas separations,Indian patent applied(2006).[11]N.Saffaj,S.A.Younsi,A.Albizane,A.Messouadi,M.Bouhria,M.Persin,M.Cretin,rbot,Elaboration and properties of TiO2–ZnAl2O4ultrafiltration membranes deposited on cordierite support,Sep.Purif.Technol.36(2004) 107–114.[12]N.Saffaj,S.A.Younsi,M.Persin,M.Cretin,A.Albizane,rbot,Processingand characterization of TiO2/ZnAl2O4ultrafiltration membranes deposited on tubular support prepared from Moroccan clay,Ceram.Int.31(2005)205–210.[13]J.K.Shim,I.K.Yoo,Y.M.Lee,Design and operation considerations for wastewatertreatment using aflat submerged membrane bioreactor,Process Biochem.38 (2002)279–285.[14]P.Le.Clech,B.Jefferson,I.S.Chang,S.J.Judd,Criticalflux determination by theflux-step method in a submerged membrane bioreactor,J.Membr.Sci.227 (2003)81–93.[15]L.S.Clesceri,A.E.Greenberg,A.D.Eaton,Standard Methods for the Examina-tion of Water and Wastewater,20th ed.,American Public Health Association, Washington,1998.[16]I.S.Chang,C.H.Lee,Membranefiltration characteristics in membrane coupledactivated sludge system—the effect of physiological states of activated sludge on membrane fouling,Desalination120(1998)221–233.[17]O.H.Lowry,N.J.Rosebrough,L.Farr,R.J.Randall,Protein measurement with thefolin phenol reagent,J.Biol.Chem.193(1951)265–275.[18]M.Dubois,K.A.Gilles,J.K.Hamilton,P.A.Rebers,F.Smith,Colorimetric methodfor determination of sugars and related substances,Anal.Chem.28(1956) 350–356.[19]F.Fan,H.Zhou,H.Husain,Identification of wastewater sludge characteristics topredict criticalflux for membrane bioreactor processes,Water Res.40(2006) 205–212.[20]P.Le-Clech,B.Jefferson,S.J.Judd,Impact of aeration,solids concentration andmembrane characteristics on the hydraulic performance of a membrane biore-actor,J.Membr.Sci.218(2003)117–129.[21]P.Le-Clech,V.Chen,T.A.G.Fane,Fouling in membrane bioreactor used inwastewater treatment,J.Membr.Sci.284(2006)17–53.[22]F.Meng,S.R.Chae,A.Drews,M.Kraume,H.S.Shin,F.Yang,Recent advancesin membrane bioreactors(MBRs):Membrane fouling and membrane material, Water Res.43(2009)1489–1512.[23]G.Guglielmi,D.P.Saroj,D.Chiarani,G.Andreottola,Sub-critical fouling ina membrane bioreactor for municipal wastewater treatment:Experimentalinvestigation and mathematical modeling,Water Res.41(2007)3903–3914.[24]M.Matoˇs i´c,M.Vukovi´c,M.ˇCurlin,I.Mijatovi´c,Fouling of a hollowfibre sub-merged membrane during long termfiltration of activated sludge,Desalination 219(2008)57–65.[25]J.A.Howell,H.C.Chua,T.C.Arnot,In situ manipulation of criticalflux in asubmerged membrane bioreactor using variable aeration rates,and effects of membrane history,J.Membr.Sci.242(2004)13–19.[26]S.S.Madaeni,A.G.Fane,D.E.Wiley,Factors influencing criticalflux in membranefiltration of activated sludge,J.Chem.Technol.Biotechnol.74(1999)539–543.[27]S.Rosenberger,M.Kraume,Filterability of activated sludge in membrane biore-actor,Desalination146(2002)373–379.[28]A.M.Pitt,The nonspecific protein binding of polymeric microporous mem-branes,J.Parenter.Sci.Technol.41(1987)110–113.[29]I.S.Chang,S.N.Kim,Wastewater treatment using membranefiltration—effectof biosolids concentration on cake resistance,Process Biochem.40(2005) 1307–1314.[30]L.Defrance,M.Y.Jaffrin,B.Gupta,P.Paullier,V.Geaugey,Contribution of variousconstituents of activated sludge to membrane bioreactor fouling,Bioresour.Technol.73(2000)105–112.[31]S.Rosenberger,U.Kruger,R.Witzig,W.Manz,U.Szewzyk,M.Kraume,Perfor-mance of a bioreactor with submerged membranes for aerobic treatment of municipal wastewater,Water Res.36(2002)413–420.[32]B.Fan,X.Huang,Characteristics of a self-forming dynamic membrane coupledwith a bioreactor for municipal wastewater treatment,Environ.Sci.Technol.36(2002)5245–5251.。