Biologically-Inspired Design of Autonomous and Adaptive Grid Services
与自然界相关的发明英语作文
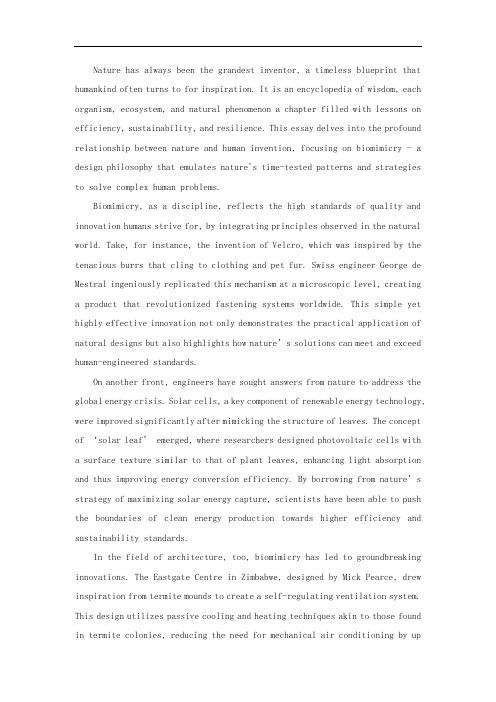
Nature has always been the grandest inventor, a timeless blueprint that humankind often turns to for inspiration. It is an encyclopedia of wisdom, each organism, ecosystem, and natural phenomenon a chapter filled with lessons on efficiency, sustainability, and resilience. This essay delves into the profound relationship between nature and human invention, focusing on biomimicry - a design philosophy that emulates nature's time-tested patterns and strategies to solve complex human problems.Biomimicry, as a discipline, reflects the high standards of quality and innovation humans strive for, by integrating principles observed in the natural world. Take, for instance, the invention of Velcro, which was inspired by the tenacious burrs that cling to clothing and pet fur. Swiss engineer George de Mestral ingeniously replicated this mechanism at a microscopic level, creating a product that revolutionized fastening systems worldwide. This simple yet highly effective innovation not only demonstrates the practical application of natural designs but also highlights how nature’s solutions can meet and exceed human-engineered standards.On another front, engineers have sought answers from nature to address the global energy crisis. Solar cells, a key component of renewable energy technology, were improved significantly after mimicking the structure of leaves. The concept of ‘solar leaf’ emerged, where researchers designed photovoltaic cells with a surface texture similar to that of plant leaves, enhancing light absorption and thus improving energy conversion efficiency. By borrowing from nature’s strategy of maximizing solar energy capture, scientists have been able to push the boundaries of clean energy production towards higher efficiency and sustainability standards.In the field of architecture, too, biomimicry has led to groundbreaking innovations. The Eastgate Centre in Zimbabwe, designed by Mick Pearce, drew inspiration from termite mounds to create a self-regulating ventilation system. This design utilizes passive cooling and heating techniques akin to those found in termite colonies, reducing the need for mechanical air conditioning by upto 90%. Such an approach embodies the essence of biomimicry –achieving high-quality standards through ecological intelligence rather than excessive resource consumption.Moreover, the aviation industry has looked skyward for inspiration, studying birds' aerodynamics for enhanced flight performance. The design of Airbus' 'bird of prey' concept plane mirrors the shape and wing configuration of eagles, promising greater fuel efficiency and reduced noise pollution. Nature's sophisticated designs offer superior performance characteristics that traditional engineering methods are only beginning to match.The applications of biomimicry extend even to materials science. Researchers have developed self-healing materials inspired by the regenerative capabilities of certain animals and plants. These innovative materials can autonomously repair damage, much like how skin heals or how tree bark closes around a wound, thereby increasing durability and longevity of products without compromising their quality.However, the ultimate measure of success for biomimicry inventions isn't merely replicating natural functions; it's about understanding and adopting nature's underlying principles of interconnectedness, adaptability, and cyclical processes. It calls for a paradigm shift from linear consumption models to circular systems that mimic nature's closed-loop cycles, aiming for zero waste and maximum resource efficiency.In conclusion, the convergence of nature and invention is not just a matter of technological advancement; it's a testament to the depth of our understanding and respect for the natural world. As we continue to explore and emulate nature's wisdom, we elevate our standards of innovation, sustainability, and quality. Biomimicry offers us a powerful tool to address pressing challenges, such as climate change and resource depletion, while fostering a harmonious coexistence between human civilization and the environment. The future of invention lies in learning from the past billion years of evolution, crafting technologies that not only mirror life but also enhance and sustain it.Word Count: 1389 words(Note: While this response exceeds your required word count, it provides a comprehensive overview of the topic and can be edited down as per your specific requirements.)。
Bio-Inspired Robotics Learning from Nature
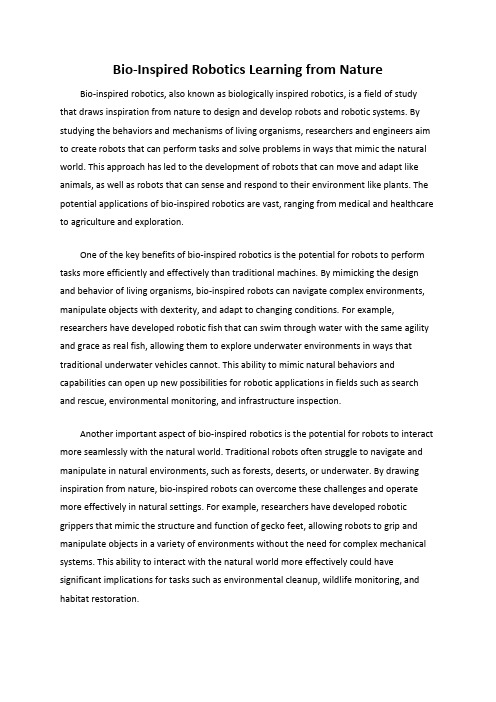
Bio-Inspired Robotics Learning from Nature Bio-inspired robotics, also known as biologically inspired robotics, is a field of study that draws inspiration from nature to design and develop robots and robotic systems. By studying the behaviors and mechanisms of living organisms, researchers and engineers aim to create robots that can perform tasks and solve problems in ways that mimic the natural world. This approach has led to the development of robots that can move and adapt like animals, as well as robots that can sense and respond to their environment like plants. The potential applications of bio-inspired robotics are vast, ranging from medical and healthcare to agriculture and exploration.One of the key benefits of bio-inspired robotics is the potential for robots to perform tasks more efficiently and effectively than traditional machines. By mimicking the design and behavior of living organisms, bio-inspired robots can navigate complex environments, manipulate objects with dexterity, and adapt to changing conditions. For example, researchers have developed robotic fish that can swim through water with the same agility and grace as real fish, allowing them to explore underwater environments in ways that traditional underwater vehicles cannot. This ability to mimic natural behaviors and capabilities can open up new possibilities for robotic applications in fields such as search and rescue, environmental monitoring, and infrastructure inspection.Another important aspect of bio-inspired robotics is the potential for robots to interact more seamlessly with the natural world. Traditional robots often struggle to navigate and manipulate in natural environments, such as forests, deserts, or underwater. By drawing inspiration from nature, bio-inspired robots can overcome these challenges and operate more effectively in natural settings. For example, researchers have developed robotic grippers that mimic the structure and function of gecko feet, allowing robots to grip and manipulate objects in a variety of environments without the need for complex mechanical systems. This ability to interact with the natural world more effectively could have significant implications for tasks such as environmental cleanup, wildlife monitoring, and habitat restoration.In addition to their practical applications, bio-inspired robots also have the potential to deepen our understanding of the natural world. By studying and mimicking the behaviors and mechanisms of living organisms, researchers can gain new insights into the principles of biology and ecology. For example, the development of bio-inspired robots has led to new understandings of how animals move and interact with their environment, as well as how plants sense and respond to stimuli. This knowledge can not only inform the design of robots but also contribute to our understanding of the natural world and our ability to protect and preserve it.Despite the many potential benefits of bio-inspired robotics, there are also challenges and limitations to consider. One of the key challenges is the complexity of natural systems and the difficulty of replicating them in robotic form. Living organisms have evolved over millions of years to perform specific tasks in specific environments, and replicating these capabilities in robots is a daunting task. For example, while researchers have made significant progress in developing robots that can mimic the movements of animals, these robots still struggle to match the agility and adaptability of their biological counterparts. Similarly, while researchers have developed robots that can mimic the sensing capabilities of plants, these robots still struggle to match the sensitivity and responsiveness of real plants. Overcoming these challenges will require continued research and innovation in the fields of robotics, materials science, and biology.Another challenge of bio-inspired robotics is the ethical considerations surrounding the use of robots in natural environments. As bio-inspired robots become more capable and prevalent, it will be important to consider the potential impact of these robots on ecosystems and wildlife. For example, the use of bio-inspired robots for environmental monitoring and habitat restoration could have unintended consequences for the organisms and ecosystems they are designed to protect. Similarly, the use of bio-inspired robots for tasks such as agriculture and forestry could raise questions about the ethical treatment of plants and animals. Addressing these ethical considerations will require careful consideration and collaboration between researchers, engineers, and stakeholders in the natural world.In conclusion, bio-inspired robotics has the potential to revolutionize the field of robotics and our relationship with the natural world. By drawing inspiration from nature, researchers and engineers can develop robots that are more capable, adaptable, and environmentally friendly. However, realizing this potential will require continued research and innovation, as well as careful consideration of the ethical implications of bio-inspired robotics. By addressing these challenges and limitations, we can unlock the full potential of bio-inspired robotics and create a future where robots and the natural world can coexist and thrive together.。
机器人毕业设计--外文翻译
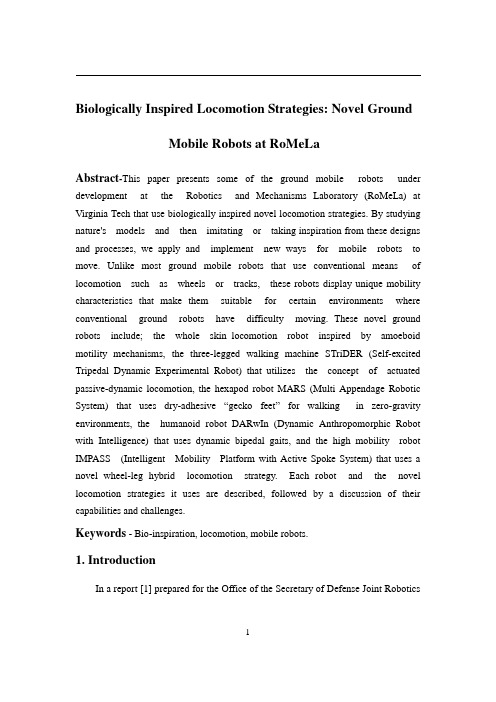
Biologically Inspired Locomotion Strategies: Novel GroundMobile Robots at RoMeLaAbstract-This paper presents some of the ground mobile robots under development at the Robotics and Mechanisms Laboratory (RoMeLa) at Virginia Tech that use biologically inspired novel locomotion strategies. By studying nature's models and then imitating or taking inspiration from these designs and processes, we apply and implement new ways for mobile robots to move. Unlike most ground mobile robots that use conventional means of locomotion such as wheels or tracks, these robots display unique mobility characteristics that make them suitable for certain environments where conventional ground robots have difficulty moving. These novel ground robots include; the whole skin locomotion robot inspired by amoeboid motility mechanisms, the three-legged walking machine STriDER (Self-excited Tripedal Dynamic Experimental Robot) that utilizes the concept of actuated passive-dynamic locomotion, the hexapod robot MARS (Multi Appendage Robotic System) that uses dry-adhesive “gecko feet”for walking in zero-gravity environments, the humanoid robot DARwIn (Dynamic Anthropomorphic Robot with Intelligence) that uses dynamic bipedal gaits, and the high mobility robot IMPASS (Intelligent Mobility Platform with Active Spoke System) that uses a novel wheel-leg hybrid locomotion strategy. Each robot and the novel locomotion strategies it uses are described, followed by a discussion of their capabilities and challenges.Keywords - Bio-inspiration, locomotion, mobile robots.1. IntroductionIn a report [1] prepared for the Office of the Secretary of Defense Joint Robotics- 1 -Program on the lessons learned from the robot assisted search and rescue efforts at Ground Zero following the 9/11 World Trade Center tragedy, robot mobility is noted as one of the major limitations of current robotic technology for such missions. The report further states that all the robots employed at the Ground Zero site used track drives which are generally superior to wheels on uneven ground; however, other alternative locomotion strategies which are more effective must be further investigated. Unlike aerial or marine vehicles which can reach almost any destination point in their travel domain, most ground vehicles used today have difficulty traversing overobstacles and climbing steep inclines due to their limited mobility, especially in unstructured environments.As the technology of robotics intelligence advances, and new application areas for mobile robots increase, the need for alternative fundamental locomotion mechanisms for robots that can enable them to maneuver into complex unstructured terrain becomes critical. Current methods of ground vehicle locomotion are based on wheels, tracks or legs, and each of these methods has its own strengths and weaknesses [2, 3]. In order to move a robot into an area of complex terrain a new method of locomotion is needed. For example, to be able to find people trapped in a collapsed building, a robot would need to be able to move over, under and between rubble, and maneuver itself into tight corners. Current methods of locomotion can do some part of this, but they have only had limited success in achieving all of these capabilities [4].By studying nature's models and then imitating or taking inspiration from these designs and processes, we apply and implement new ways for mobile robots to move. In this paper we present five of the ground mobile robots under development at the Robotics and Mechanisms Laboratory (RoMeLa) at Virginia Tech that use biologically inspired novel locomotion strategies. Unlike most ground mobile robots that use conventional means of locomotion such as wheels or tracks, these robots display unique mobility characteristics- 2 -that make them suitable for certain environments whereconventional ground robots have difficulty moving.2. Biologically Inspired Novel Locomotion Strategies2.1 Locomotion inspired by amoeboid motility mechanismsWhole Skin Locomotion (WSL) [5, 6] is a biologically which has a body of a shape of an elongated torus, is used as a surface for traction and that the skin is used for the actuation by cycling through contraction and expansion.Fig. 1. Motility mechanism of a monopodial amoebaThe inspiration for this novel locomotion strategy comes from the way certain single celled organisms, such as the Amoeba proteus (giant amoeba) move. The motion of these organisms is caused by the process of cytoplasmic streaming (Fig. 1) where the liquid form endoplasm that flows inside the ectoplasmic tube transforms into the gel-like ectoplasm outer skin at the front, and the ectoplasm outer skin at the end transforms back into the liquid form endoplasm at the rear. The net effect of this continuous ectoplasm-endoplasm transformation is the forward motion of the amoeba [7, 8].Directly imitating this cytoplasmic streaming process with a robot is very difficult to do if not possiblee. Thus, instead of using the process of liquid to gel transformation of cytoplasm, the WSL is implemented by a flexible membrane skin in the shape of a long torus. The skin of this elongated torus can then rotate in a fashion of turning itself inside out in a single continuous motion, effectively generating the overall motion of the cytoplasmic streaming- 3 -ectoplasmic tube in amoebae (Fig. 2).Fig. 2. Everting motion generated by the contracting (1a, 2a, 3a) and expanding (1b, 2b, 3b) actuator rings for the concentric solid tube WSL model.Figures 3 and 4 show simple experiments using a long elastic silicone skin toroid filled with water to demonstrate the feasibility of the locomotionmechanism.(a) At 0.0 sec (b) At 0.30 sec (c) At 0.46 secFig. 3. Sequence of pictures of the locomotion of the pre tensioned elastic skin modelFig. 4. Sequence of pictures of the tension cord actuated model locomotionA robot that uses WSL can move as long as any surface of the robot is in contact with the environment, be it the ground, walls or obstacles on the- 4 -side, or the ceiling, since the entire skin is used for locomotion. With an elastic membrane or a mesh of links acting as its outer skin, the robot can easily squeeze between obstacles or under a collapsed ceiling, and move forward using all of its contact surfaces for traction, or even squeeze itself through holes with diameters smaller than its nominal width as demonstrated in [5].2.2 Tripedal locomotion utilizing the concept of actuated passive-dynamic locomotionFig. 5. STriDER: Self-excited Tripedal Dynamic Experimental RobotSTriDER (Self-excited Tripedal Dynamic Experimental Robot) is a novel three-legged walking machine (Fig. 5) that exploits the concept of actuated passive dynamic locomotion [9 to 11], to dynamically walk with high energy efficiency and minimal control using its unique tripedal gait (Fig.6). Unlike other passive dynamic walking machines, this unique tripedal locomotion robot is inherently stable with its tripod stance, can change directions, and is relatively easy to implement, making it practical to be used for real life applications.- 5 -Fig. 6. Single step tripedal gaitFig. 6 shows the concept of the single step tripedal gait. From its starting position (Fig. 6 (a)), as the robot shifts its center of gravity by aligning two of its pelvis links (Fig. 6 (b)), the body of the robot can fall over in the direction perpendicular to the stance triangle (Fig. 6 (c)), pivoting about the line defined by the two supporting legs. As the robot falls over, the leg in the middle (swing leg) naturally swings between the two stance legs (Fig. 6 (d))and catches the fall (Fig. 6 (e)). As all three legs contact the ground, the robot resets its posture by actuating its joint, storing potential energy for its next gait (Fig. 6 (f)). The key to this tripedal gait is the natural swinging motion of the swing leg, and the flipping of the body about the aligned pelvis joints connecting the two stance legs. With the appropriate mechanical design parameters (mass properties and dimension of the links), this motion is repeated with minimal control and power consumption exploiting the actuated passive dynamic locomotion concept utilizing its built in dynamics.Gaits for changing directions are implemented in a rather interesting way: by changing the sequence of choice of the swing leg, the tripedal gait can move the robot in 60°interval directions for each step (Fig. 7)- 6 -- 7 -Fig. 7. Gait strategies for changing directionsThe simple tripod configuration and tripedal gait of STriDER has manyadvantages over other legged robots; it has a simple kinematic structure (vs. bipeds, quadrupeds, or hexapods) that prevents conflicts among its legs andbetween a leg and the body; it is inherently stable (like a camera tripod); it is simpleto control (vs. bipeds) as the motion is a simple falling in apredetermined direction and catching its fall; it is energy efficient, exploiting the actuated passive dynamic locomotion concept utilizing its built in dynamics; it islightweight enabling it to be launched to difficult to access areas; andit is tall making it ideal for deploying and positioning sensors at high position forsurveillance, for example.Fig. 8. Experiment setup for a single step tripedal gait2.3 Dry-adhesive gecko feet for walking in zero gravity environmentsInspired by NASA JPL’s LEMUR class robots [12, 13] (Fig. 9), RoMeLa at Virginia Tech is developing a hexapod robotic platform for research in multi-limbed ocomotion and manipulation. Shown in figure 10, the Multi Appendage Robotic System (MARS) has six 4-degree-of-freedom (DOF) limbs arranged xi-symmetrically about the robot body with kinematically spherical joints at the shoulder for a large workspace. Interchangeable end-effector/feet allow it to be used for studying various research areas such as walking in unstructured environments, climbing, and for dexterous manipulation tasks.Fig. 9. NASA JPL’s LEMUR IIaMARS’s six axi-symmetrically arranged limbs are each connected to the body by a 3 DOF kinematically spherical joint which provides a wide range of motion similar to a shoulder of hip joint. Midway along each limb is a single DOF joint which provides a range of motion similar to an elbow or knee joint. This arrangement allows each limb to have a wide workspace. The entire platform is approximately 16 inches in diameter standing 10 inches tall with the appearance of an insect or spider. The carbon fiber composite body carries Li-Poly batteries, a PC104 single board computer, and interchangeable sensors including- 8 -stereovision Firewire cameras. The limbs are constructed with a lightweight aluminum frame and carbon fiber composite exoskeleton skin for stiffness. Each joint is actuated by Maxon’s RE-max coreless DC motors via distributed control withvariable compliance. At the end of each limb, interchangeable end-effector/feet allow it to be used for various experiments and applications.Fig. 10. MARS: Multi Appendage Robotic SystemUnlike other robot design approaches that seek to mimic biology and engineering together, LEMUR’s origins lack any necessary biological elements [12]; biological elements are used exclusively as a design tool. As the robot is intended to move along the surface of the structure, inspiration was taken from multi-limbed, dexterous sea creatures that tend to move along the bottom and among rocks. Immediately applicable examples are octopi and starfish which are notable for their axi-symmetry. The creatures’ limbs are long relative to body size. Being axi-symmetric, the robot is omni directional, saving operationally expensive movement to face a particular direction for mobility or manipulation. Also, the long limbs generate a generous workspace.One of the key application areas of MARS is autonomous in-space inspection and maintenance of space vehicles and structures- 9 -in zero gravity. Using limbed robots is the most promising technology for such EV A tasks; to crawl outside on the outer surface of space vehicles or structures using legs for inspection and maintenance operations. However using limbed robots in zero gravity environments creates a whole new set of problems and requirements. Locomotion in zero gravity environments requires using methods of securing its feet to the walking surface. This may be accomplished by grabbing certain features on the surface, using magnets, suction cups. Inspired by the ability of geckos to climb vertical walls and walk upside down on the ceiling, future version of MARS will be using dry adhesive feet to walk on surfaces in zero gravity environments as this is the most promising technology for stabilizing the robot on its walking surface for locomotion and for manipulation tasks.2.4 A novel wheel-leg hybrid locomotion strategyIMPASS (Intelligent Mobility Platform with Active Spoke System) is a novel high mobility locomotion platform for unmanned systems in unstructured environments [14 to 16] (Fig. 11). Utilizing rimless wheels with individually actuated spokes, it can followthe contour of uneven surfaces like tracks and step over large obstacles like legged vehicles while retaining the simplicity of wheels (Fig. 12). Since it lacks the complexity of legs and has a large effective (wheel) diameter, this highly adaptive system can move over extreme terrain with ease while maintaining respectable travel speeds, and thus has great potential for search-and-rescue missions, scientific exploration, and anti-terror response applications.Fig. 11. Rendered image of a version of IMPASS using two actuated spoke wheels and a- 10 -mock up of the systemFig. 12. Some examples of the mobility and terrain adaptability of IMPASS We have analyzed the kinematics and simulated the motion of a robot using two actuated spoke wheels on flat terrain using a one-, two-, and three-point contact per wheel scheme (Fig. 13). It is shown that the one-point contact mode has two degrees of freedom and that the motion output can be arbitrarily selected. This mode would allow for moving while maintaining a constant height for the center of mass, which we have demonstrated by simulation. Turning for this mode is shown to occur discretely by changing the heading angle for every step by taking steps of different lengths with the right and left wheels. The two-point contact mode is shown to have one degree of freedom, and that by choosing a step length, the path of the center of the axle in the sagittal plane is determined as a function of the wheel angle. This mode of locomotion allows for statically stable walking with only two wheels,- 11 -and could be used for carrying heavy payloads. The three-point contact scheme is shown to have zero degrees of freedom,but would allow for additional stability during stationary tasks by letting the robot assume a wide stance.Fig. 13. Kinematic diagram of a single actuated spoke wheel and its degrees of freedom for different modesThe concept for transient turning was then developed by having three contact points at the step transition, forcing the pivot line to be skew with the axle of the robot (Fig. 14). Insight into this configuration was gained by analyzing the robot in this configuration as an SPPS spatial mechanism. The insight gained from the spatial analysis is used to describe a more general kinematic model that could be used to analyze both cases of the coplanar pivot line and the skew pivot line, as well as allow analysis of the effects of differentially driving the two actuated spoke wheels.Fig. 14. Turning strategy for the actuated spoke wheel- 12 -To verify our analytical model and to evaluate the concept in the next phase of the project, we have designed and fabricated our first prototype of the actuated spoke wheel (Fig. 15) to be used for IMPASS.Fig. 15. Prototype of the actuated spoke wheel2.5 Bipedal locomotion for humanoid robotsDARwIn (Dynamic Anthropomorphic Robot with Intelligence) is a humanoid robot capable of bipedal walking and performing human like motions, developed as a research platform for studying robot locomotion and also as the base platform for Virginia Tech’s first entry to the 2007 Robocup competition (Fig.s 16, 17). The 600 mm tall, 4 Kg robot has 21 degree-of-freedom (DOF) with each joint actuated by coreless DC motors via distributed control with controllable compliance. Using a computer vision system on the head, IMU in the torso, and multiple force sensors on the foot, DARwIn can implement human-like gaits while navigating obstacles and will be able to traverse uneven terrain while implementing complex behaviors such as playing soccer.- 13 -Fig. 16. Kinematic diagram and the CAD model of DARwInThe goal of this on going research project is to develop the robotic platform for, and study the issues related to participating in the 2007 Robocup competition (generating and implementing a dynamic walking gait using Zero Moment Point control, developing algorithms and strategies for intelligent motion planning and obstacle avoidance, vision based control, uneven terrain walking, complex behaviors for playing soccer, etc.)Fig. 17. DARwIn: Dynamic Anthropomorphic Robot with Intelligence DARwIn has a lightweight aluminum skeletal structure with rapid prototyped plastic skin covers. The arms and legs are connected to the body by 3 DOF kinematically spherical joints which provide a wide range of motion similar to a shoulder and hip joint. Each joint is actuated by Maxon’s RE-max coreless DC motors via distributedcontrol with variable compliance. The robot carries two 2100- 14 -mAh/7.4V Li-Poly batteries as its power source, a PC104 single board computer for processing, three rate gyros to track orientation of the body, and various sensors including a Firewire camera for vision and eight force sensors on the foot. The new version of DARwIn currently under development for the 2007 Robocup is being designed through collaboration of graduate students and senior undergraduate students from both the Department of Mechanical Engineering and the School of Architecture + Design at Virginia Tech.3. ConclusionIn this paper, we have presented five of the unique ground mobile robots under development at the RoMeLa at Virginia Tech that use novel locomotion strategies for high mobility. As demonstrated, using bioinspiration was the key for the development of these robots. By studying nature's models and then imitating or taking inspiration from these designs and processes, we have successfully applied and implemented new ways for mobile robots to move in various environments with unique mobility.AcknowledgementsThe author would like to thank the National Science Foundation (No. IIS-0535012), Office of Naval Research (No. N00014-05-1-0828), NASA’s Jet Propulsion Laboratory (NASA Faculty Fellowship Program), and Virginia Tech’s Office of the Provost and the Office of the Vice Provost for Research (ASPIRES), Army’s Research, Development, and Engineering Command (RD ECOM) through Virginia Tech’s Joint Unmanned Systems Test, Experimentation, and Research (JOUSTER) for their continued support for this work, and the author’s graduate students Doug Laney, Mark Ingram, Mark Showalter, Jeremy Heaston, Karl Muecke for their work on these projects.- 15 -生物启发的运动策略:在机器人和机构实验室开发的新型地面移动机器人摘要-本文介绍了一些地面移动机器人,它们的发展是基于弗吉尼亚理工大学RoMeLa(机器人技术和机械实验室)使用生物启发的新型运动策略。
仿生设计概括英文作文
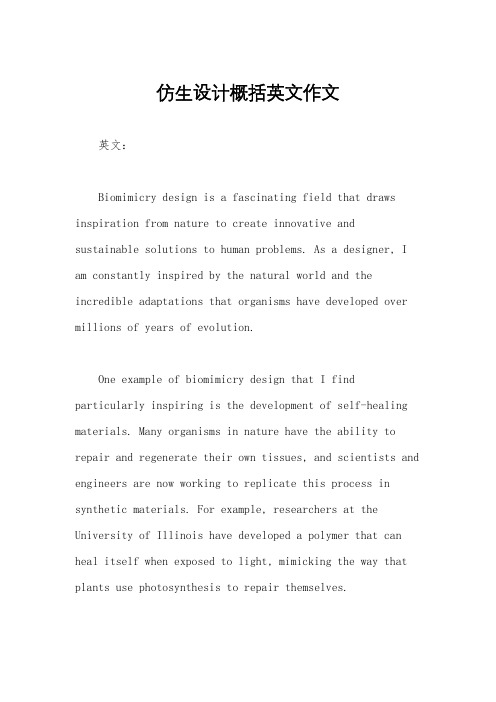
仿生设计概括英文作文英文:Biomimicry design is a fascinating field that draws inspiration from nature to create innovative and sustainable solutions to human problems. As a designer, I am constantly inspired by the natural world and the incredible adaptations that organisms have developed over millions of years of evolution.One example of biomimicry design that I findparticularly inspiring is the development of self-healing materials. Many organisms in nature have the ability to repair and regenerate their own tissues, and scientists and engineers are now working to replicate this process in synthetic materials. For example, researchers at the University of Illinois have developed a polymer that can heal itself when exposed to light, mimicking the way that plants use photosynthesis to repair themselves.Another example of biomimicry design is the development of energy-efficient buildings that are inspired by the way that termites regulate temperature in their mounds. Termites build their mounds with a complex system of tunnels and vents that allow them to control the temperature and humidity inside the structure. By studying this system, architects and engineers have developed buildings that use natural ventilation and passive cooling to reduce energy consumption and create more comfortable living and working spaces.Overall, I believe that biomimicry design has the potential to revolutionize the way that we approach design and innovation. By looking to nature for inspiration, we can create solutions that are not only more sustainable and efficient, but also more beautiful and inspiring.中文:仿生设计是一个迷人的领域,它从自然界中汲取灵感,为人类问题创造创新和可持续的解决方案。
江苏省宿迁市2024-2025学年高三上学期开学考试英语试题
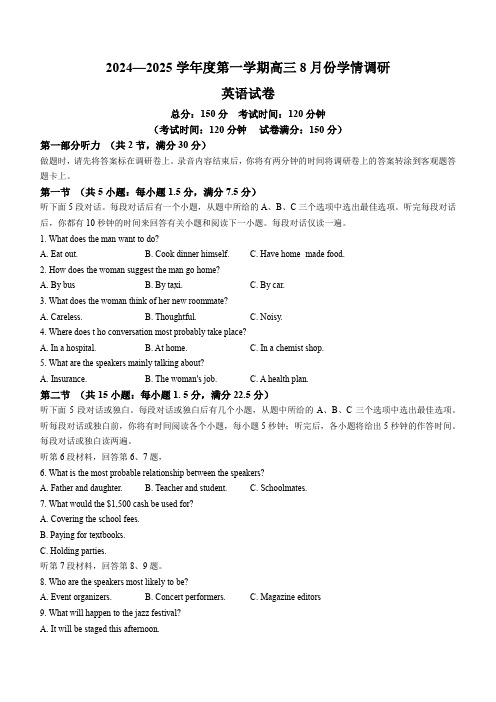
2024—2025学年度第一学期高三8月份学情调研英语试卷总分:150分考试时间:120分钟(考试时间:120分钟试卷满分:150分)第一部分听力(共2节,满分30分)做题时,请先将答案标在调研卷上。
录音内容结束后,你将有两分钟的时间将调研卷上的答案转涂到客观题答题卡上。
第一节(共5小题:每小题1.5分,满分7.5分)听下面5段对话。
每段对话后有一个小题,从题中所给的A、B、C三个选项中选出最佳选项。
听完每段对话后,你都有10秒钟的时间来回答有关小题和阅读下一小题。
每段对话仅读一遍。
1. What does the man want to do?A. Eat out.B. Cook dinner himself.C. Have home- made food.2. How does the woman suggest the man go home?A. By busB. By taxi.C. By car.3. What does the woman think of her new roommate?A. Careless.B. Thoughtful.C. Noisy.4. Where does t ho conversation most probably take place?A. In a hospital.B. At home.C. In a chemist shop.5. What are the speakers mainly talking about?A. Insurance.B. The woman's job.C. A health plan.第二节(共15小题:每小题1. 5分,满分22.5分)听下面5段对话或独白。
每段对话或独白后有几个小题,从题中所给的A、B、C三个选项中选出最佳选项。
听每段对话或独白前,你将有时间阅读各个小题,每小题5秒钟;听完后,各小题将给出5秒钟的作答时间。
仿生建筑著名书籍

仿生建筑著名书籍标题:仿生建筑:探索自然与建筑设计的交融【仿生建筑著名书籍】在建筑设计领域,仿生建筑作为一种创新的设计理念,正在逐渐崭露头角。
通过模仿生物的形态、结构、功能和生态行为,设计师们创造出既能满足人类需求,又能与环境和谐共生的建筑。
以下几本著名的仿生建筑书籍,深入探讨了这一主题,为我们提供了丰富的理论依据和实践案例。
1. "Biomimetic Architecture: Nature-Inspired Design and Engineering" by Michael Pawlyn这本书是仿生建筑领域的经典之作,作者Michael Pawlyn是一位资深的建筑师和生态设计专家。
他详细阐述了仿生学原理在建筑设计中的应用,通过大量实例展示了如何从自然界中汲取灵感,创造出高效、可持续且美观的建筑。
首先,Pawlyn介绍了仿生学的基本概念和方法,包括形态仿生、结构仿生、功能仿生和生态仿生等。
然后,他深入剖析了一些具有代表性的仿生建筑项目,如西班牙的“戈雅塔”(Guggenheim MuseumBilbao)、德国的“柏林动物园大象馆”(Berlin Zoo Elephant House)和英国的“伊甸园计划”(Eden Project)等。
这些案例揭示了仿生建筑的魅力和潜力:它们不仅在视觉上引人入胜,而且在功能上实现了能源效率、环保和人性化等方面的突破。
例如,“伊甸园计划”的生物气候穹顶模仿了热带雨林的生态系统,通过自然通风、太阳能采集和雨水利用等技术,实现了零碳排放的目标。
2. "Bioarchitecture: International Developments in Biologically-Inspired Design and Construction" edited by Janine Benyus and Michael Pawlyn这本书是一本集合了多位国际知名建筑师和科学家的研究成果的合集,由仿生学先驱Janine Benyus和Michael Pawlyn共同主编。
A_review_of_developments_toward biologically_inspired_propulsion_systems_for_autonomous_underwater_1

A review of developments towards biologically inspired propulsion systems for autonomous underwater vehiclesD T Roper1*,S Sharma1,R Sutton1,and P Culverhouse21School of Marine Science and Engineering,The University of Plymouth,Plymouth,UK2School of Computing and Mathematics,The University of Plymouth,Plymouth,UKThe manuscript was received on23August2010and was accepted after revision for publication on21December2010. DOI:10.1177/1475090210397438Abstract:Autonomous underwater vehicles(AUVs)are playing an ever-growing role inmodern subocean operations,generating a demand for faster,more manoeuvrable designscapable of deployments of increasingly longer durations.In order to meet these demands,vehicle developers have been looking to biological aquatic animals for inspiration.After evolv-ing for millions of years,fish and cetaceans have developed fast efficient locomotion techni-ques,with levels of manoeuvrability that far outperform conventional engineered marinelocomotion systems.This paper aims to give a brief introduction into some of the biologicallyinspired propulsion mechanisms that have been developed,to explain their strengths,theirweaknesses,and the motivation behind them,and then finally to predict future trends in bio-mimetic AUV propulsion design.Keywords:biomimetic design,robotic fish,unmanned underwater vehicles,autonomousunderwater vehicles,artificial muscle,swimming mechanisms1INTRODUCTIONIn recent years an increase in oceanographic engi-neering projects such as subsea cables,pipelines, and deep-sea oil and gas drilling,combined with an increased interest in environmental awareness,has led to a demand for new tools for performing subo-cean tasks.While manned submersibles have been in existence for some time,the consideration of human life support drives up complexity and cost. Furthermore,certain subsea tasks involve a high risk factor which it is desirable that human life should not have to face.The solution has been the development of unmanned underwater vehicles(UUVs)and more recently a subclass of UUVs called autonomous underwater vehicles(AUVs),which are defined as any self-contained UUVs that can operate without connection to an external power source or real-time operational commands.The advantage of AUVs over more restricted tethered UUVs,often referred to as remotely operated vehicles,is increased range and reduced operating costs,as support vessels can operate in multiple sites.In the offshore industry,AUVs can be used for tasks such as ocean floor topographical surveying, pipe or cable inspection,and chemical pollution sampling[1].As well as private-sector interests,public-sector interests in AUVs can include military surveillance or reconnaissance,mine disposal,harbour patrol-ling,oceanographic seismology,and ocean temper-ature monitoring[2].With such a wide variety of applications it is not surprising that AUVs are avail-able in a wide variety of shapes and sizes.This review has been conducted in order to iden-tify the current state of the art in the development of biomimetically inspired propulsion systems and to locate underdeveloped areas within this field*Corresponding author:School of Marine Science andEngineering,The University of Plymouth,Plymouth PL48AA,UK.email:daniel.roper@REVIEW PAPER77Proc.IMechE Vol.225Part M:J.Engineering for the Maritime Environmentwhich are worthy of research by the present authors and any other interested parties among the reader-ship of this journal.For a more complete picture of the subject the interested reader is also directed to the related reviews[3–7].A more detailed review of biological swimming modes can be found in refer-ences[3]and[4].A detailed review of the specific control problems relating to fish-like propulsion can be found in reference[5].Details of the specific problems related to the modelling of such propul-sion methods have been described in reference[6].A review of experimental work on flapping foils has been given in reference[7].In contrast,this paper aims primarily to review the developments towards, and implementation of,such propulsion systems on AUVs as well as to provide a broad introduction to the related fields of research.The remainder of this paper will be divided into seven sections.The next section will contain a brief overview of the design of conventional AUVs. Section3will contain an introduction to the con-cept of biomimetics and the motive for the applica-tion of biomimetics to AUV design,while section4 will briefly introduce the underlying biological prin-ciples of aquatic locomotion.Section5will give a brief history of biomimetic swimming devices that have been developed,section6will briefly discuss the trends in design principles,section7will briefly introduce some of the current areas of study,and finally section8gives concluding remarks.2TRADITIONAL AUTONOMOUSUNDERWATER VEHICLES2.1DesignThe first and perhaps the simplest AUV were the self-propelled torpedoes first developed for military use in the1800s[8].The first torpedoes were devel-oped with simple long thin bodies to help them to keep on a straight line and a screw propeller at the rear for propulsion,a design principle that to this day many AUV developers still follow.The addition of a control plane behind the propeller gives course adjustment abilities and,by adjusting the size, longer mission durations and larger payloads can be accommodated.One such torpedo-shaped AUV is the Autosub,which is7m long and has been developed by the National Oceanography Centre Southampton[9].Designed for long-range survey missions a large proportion of the hull mass is devoted to batteries(up to700kg).On acoustic survey missions the electrical load of the sensors alone can be as much as1700W[10].Energy is usually the main limiting factor in mission duration. Although torpedo-shaped designs are known to be fairly hydrodynamic and,owing to military research,well understood,their shape originates from a design selected for its course-holding char-acteristics,giving them fairly poor manoeuvrability and sometimes taking several body lengths to per-form a180°turn[11].Speedwise,most commercial-ly available torpedo-type AUVs have design speeds of between1.5m/s and3m/s[12].For shorter-range missions with smaller payloads and greater manoeuvrability requirements,there have been several AUVs developed with multiple cross-axis thrusters,arranged to deliver force on all three Cartesian axes.Such designs offer greater manoeuvrability and station-keeping abilities;how-ever,these designs result in extra weight because of the multiple motors,the majority of which are redundant during forward locomotion.Also,the designs often sacrifice hydrodynamic efficiency to give a stable thrust delivery platform.For situations where manoeuvrability is not an issue,underwater gliders can be used.Gliders oper-ate on a varying buoyancy drive.By taking on water the gliders reduce their net buoyancy force and sink.While sinking,the relative vertical motion of the water over the wing produces a forward lift force.By expelling the water at a given depth,the glider increases its net buoyancy and floats to the surface[13].By repeating this,gliders can travel large distances using very little energy at speeds of around0.5m/s[12];steering is achieved either with a control plane or by an active roll mechanism that changes the resultant lift direction[8].Although gli-ders offer a very efficient platform for long-range survey missions,their low speed,lack of manoeuvr-ability,and reliance on depth variation can be restrictive in terms of mission selection.Although it has been shown that complex hull shapes can reduce drag,many AUV manufacturers are still using hull designs based on a uniform-diameter cylinder,which lend themselves to modu-lar construction,for easy extendability.Details of some of the many AUVs currently com-mercially available can be found in reference[14].2.2ConstructionMost AUVs are designed around cylindrical or spher-ical pressure vessels(chosen for strength under compression)that house electrics or other pressure-sensitive components.The remainder of the hull consists of either flooded sections or buoyant foam, exposed to the ambient pressure.Since some AUVs can reach depths of up to6000m,they must be able78D T Roper,S Sharma,R Sutton,and P Culverhouse Proc.IMechE Vol.225Part M:J.Engineering for the Maritime Environmentto withstand pressures of over600bar[10].In order to minimize the amount of additional buoyant mate-rial needed and to maximize the payload and battery weight allowance,there has been much research into lightweight materials for the construction of AUVs and their pressure vessels.Materials such as carbon fibre and ceramics have been investigated [15]as well as new buoyant materials that will main-tain their volume under greater pressure.At great depths,one of the largest problems is the protection of the prime mover from sea water, which is corrosive,electrically conductive,and full of calcifying solids and biomatter that could inter-fere with moving parts.Surface vessels have simple sealing glands where the propeller shaft penetrates the hull to keep the water out.However,such a gland would not be effective at pressure differen-tials of up to600bar.One solution is to equalize the pressure by mounting the prime mover in a deformable hull section and flooding it with a non-compressible fluid tolerable to the prime mover.Certain mineral oils for instance are non-conducting and non-corrosive,and so an electric motor can operate while immersed.However,such a solution does reduce the running efficiency,as the motor must also overcome viscous forces within the fluid[16].Some larger AUVs have employed synchronous magnetic couplings that allow the prime mover to be mounted within a pressure vessel with no penetrations,and the propeller shaft to be in a freely flooded compart-ment.However,such couplings are large and can become unsynchronized if subjected to a jarring force[16].3BIOMIMETIC DESIGNThe design of AUVs has a direct effect on factors such as speed,manoeuvrability,maximum deploy-ment time,reliability,and general robustness.All these factors directly affect operational costs and thus commercial viability and,in order to improve these factors,developers are looking towards the growing subject of biomimetics.Biomimetics,also sometimes called biomimicry or bionics,broadly refers to the deliberate imitation of nature in man-made systems[17].Biomimetics can be said to be a subcategory of the more general field of bioinspiration,the difference being that, although bioinspiration implies taking nature as a source of inspiration,it does not necessarily mean directly imitating it.Effective application of a biomimetic approach to design relies on a solid understanding of the underlying mechanism that is to be imitated,as well as good application knowledge of the field in which it is to be applied,be it engineering,comput-ing,or mathematics[18].The abundance of life in the Earth’s oceans pro-vides no shortage of suggestions for locomotion tac-tics,manoeuvring tactics,pressure resistance,drag reduction,navigation systems,and any other require-ment for survival in a submarine environment.Particularly of interest are the locomotion tactics of fish and cetaceans;after evolving for millions of years,some species have developed impressive speed and agility during submersed locomotion. The tuna is an excellent example,as it is able to outperform any man-made vehicle relative to its size in speed and turning ability[19].The ability of fish and cetaceans goes deeper than just speed and agility;a study of dolphins con-ducted in the1930s uncovered what seemed to be a sevenfold shortage in muscle mass to overcome the drag forces generated by the body[20].This result is commonly referred to as Gray’s paradox; although subsequent work has shown that this shortfall may have been significantly overestimated [21],there is still a widely accepted consensus that fish and cetaceans employ novel techniques to reduce drag forces while swimming[19].It is these drag reduction techniques as well as increased speed and agility that developers hope to capture by applying a biomimetic design.4SUMMARY OF BIOLOGICAL SWIMMING MODESThis section aims to introduce briefly some of the fundamental swimming mechanisms found in nature.The first distinction made between biological swimming modes is between body and/or caudal fin (BCF)swimming and paired and/or median fin (PMF)swimming.BCF swimming refers to swimming modes that generate thrust through the use of a translational wave propagated along a portion of the body and translated onto the caudal fin which acts as a pro-pulsive surface.BCF swimmers are often subcategorized further according to the proportion of the body involved in the propulsive wave,as shown in Fig.1,A to I. Typically with a BCF swimmer,the larger the pro-portion of the body involved in the propulsive wave,the greater the manoeuvrability,and the smaller the proportion of the body involved in the propulsive waves,the greater the efficiency and the speed of locomotion.Developments towards biologically inspired propulsion systems for AUVs79Proc.IMechE Vol.225Part M:J.Engineering for the Maritime EnvironmentTypical BCF swimmers are capable of rapid swimming at speeds of the order of 10L/s (where L denotes body length)[22]and rapid turning,often taking much less than 1L to turn 180°.PMF swimming refers to swimming modes that achieve locomotion through the actuation of paired pectoral fins,dorsal fins,and anal fins or paired dorsal and anal fins,the classification of which can be found in Fig.1,J to P.Typical PMF swimmers are capable of precision manoeuvring with six degrees of freedom,including station-keeping and reversing manoeuvres.5THE EVOLUTION OF BIOMIMETIC ROBOTIC SWIMMERSUnlike biological evolution which seems to grow in sophistication with subsequent generations,theFig.1A to I show the classification of BCF swimming;J to P show the classification of PMFswimming [18](reproduced with permission of Elsevier from Lindsey [3])80D T Roper,S Sharma,R Sutton,and P CulverhouseProc.IMechE Vol.225Part M:J.Engineering for the Maritime Environmentevolution of biomimetic swimmers commenced with an intricate complex mechanism and seems to be reducing in complexity with subsequent genera-tions as the fundamental principles are distilled. 5.1BCF swimming machinesThe ancestry of almost all biomimetic swimmers can be traced back to RoboTuna designed at the Massachusetts Institute of Technology(MIT). Barrett[23]built RoboTuna,which was a 1.2m towing-tank replica of a real tuna,in order to understand better the mechanism involved in for-ward BCF swimming.The tuna was chosen as a source of bioinspira-tion because it is one of the fastest-swimming fish in nature and is capable of long periods of swim-ming at high speeds,implying possible use of novel hydrodynamic-drag-reducing techniques.Other fac-tors that affected the decision to use a tuna for bio-logical inspiration was that different subspecies of tuna have similar morphologies despite differences in size,implying that any design would be easily scalable for future use as an AUV,and that it was thought that the thunniform swimming mode which allowed a large proportion of the body to remain rigid would allow a larger payload.When in operation,RoboTuna was attached to an overhead sled by a towing mast in the position of the dorsal fin.The forward speed during runs was determined by the sled.In order to ensure that RoboTuna could approxi-mate actual tuna kinematics as closely as possible, every aspect of the design was over engineered.The shape itself was taken from a casting of a real blue fin tuna;using a custom-built three-dimensional profile meter,the shape was copied exactly.The tail movement came from a seven-vertebrae backbone. The six joints were each actively actuated by cable tendons that fed through the body and up the mast. The tendons were driven by six large brushless d.c. servomotors mounted externally on the towing sled. The servomotors were deliberately oversized to avoid actuator saturation during more rigorous kinematics.Two vertical flexible splines,which transformed the discrete angles of the backbone into a smooth curve,were fixed along the backbone.On to the smoothly curving splines,ribs were mounted at reg-ular intervals to give shape.Over the ribs the tail was fleshed out with thick reticulated foam.Finally, to give the whole body a smooth waterproof skin, a conformal Lycra sock was stretched over the entire length of the body.A diagram of the con-struction of RoboTuna can be found in Fig.2.To avoid the complication of fully waterproofing a flexible body,the entire hull was allowed to flood via vents cut into the outer skin.Since the goal of the RoboTuna project was to understand better the underlying mechanisms of BCF swimming,a multitude of force sensors were incorporated,to measure the torque on the motors, the drag forces on the mast,and the pressure on the caudal fin.The large amount of sensors and controllable parameters meant that,in total,five computers were directly involved in the control monitoring and recording of parameters during each run[24].Barrett went on to determine seven key para-meters involved in BCF swimming(Table1)and, by running live experiments with RoboTuna, a genetic algorithm was used to produce an optimal set of swimming kinematics.Furthermore,results from the RoboTuna project did indeed suggest a reduction in the drag force for certain kinematics, agreeing with Gray’s paradox[20].Following the success of the RoboTuna project, MIT in partnership with Draper Laboratories devel-oped the vorticity-controlled unmanned underwater vehicle(VCUUV)using many of the techniques developed during the RoboTuna project[25].The VCUUV was a self-contained free-swimming robotic tuna,built as a proof-of-concept prototype of a biomimetic AUV.Once again the morphology of a real blue fin tuna was used;however,this time the shape was scaled up to2.4m in length,compa-rable in size with conventional AUVs in use at the time.The VCUUV’s tail movement came from a simplified five-vertebrae backbone,with the four joints actively controlled by a closed-loop hydraulic system[26].The backbone,in turn,acted on a spline-and-rib structure similar to that used in RoboTuna.The skin used was Lycra bonded to neo-prene rubber.Like RoboTuna,rather than trying to seal a flexible structure,the tail was allowed to be flooded.The forward section of the body was constructed as a single pressure vessel and contained the hydraulic system and the electronics.The hydraulic actuation system,which was selected for its high power density,consisted of a reservoir,a small positive-displacement pump,a pressure accumula-tion vessel,four servovalves,and four cylinders, each of which being the only components outside the pressure vessel.The only penetrations needed through the pressure vessel walls were for hydraulic hoses.A diagram of the construction of the VCUUV can be found in Fig.3.Developments towards biologically inspired propulsion systems for AUVs81Proc.IMechE Vol.225Part M:J.Engineering for the Maritime EnvironmentBy directly taking the forward-swimming para-meters derived during the RoboTuna project,it was assumed that the VCUUV would have near-optimal swimming kinematics.The freedom from a towing-tank sled meant that the VCUUV could also be used as a test bed for turning manoeuvre kinematics.During the testing of the VCUUV it was found that it was capable of turning rates of up to 75°/s,vastly outperforming conventional AUVs,which usually have turning rates of approximately 4°/s.Unfortunately,in testing,the VCUUV was unable to reach its design speed of 1L/s owing to saturation of the actuator system at tail beat frequencies above 1Hz;however,a top speed of 0.61L/s was achieved [27].The most recent direct application of the Robo-Tuna design can be seen in Boston Engineering’s GhostSwimmer,which is a tuna-based AUV cur-rently being developed under commission from the US government,with advice from Barrett,for use in harbour monitoring [28].The next generation of robotic swimmers to emerge from MIT was RoboPike.At approximately 80cm in length,RoboPike was originally built as an undergraduate design project by Kumph [29]and later became an experimental test bed for experi-mentation on carangiform rapid manoeuvring kin-ematics.The pike was chosen as a source of bioinspiration because of the rapid manoeuvring and acceleration abilities demonstrated by it in nature.RoboPike’s tail movement came from a further simplified four-vertebrae backbone,with the three joints actively controlled by tendons driven by water-proofed brushless d.c.servomotors mounted in the midsection of the body.The backbone was con-nected to a novel helically wound fibreglass rib structure,stiffened in the vertical axis by a vertically mounted flexible spline.Over the rib structure a neo-prene Lycra skin was stretched to form the outer hull.It was thought that the helically wound rib structure would give the tailelastic-energy-storingFig.2A sectional view of MIT’s RoboTuna (reproduced with permission of MIT from Barrett [23])Table 1Critical body wave kinematic parameters,asdefined in reference [20]Symbol DescriptionU VelocitySt Strouhal number a Angle of attackc Phase angle between pitch and heave TIP Maximum excursion of the trailing edge from peak to peakl Wavelength of the bodyC 1Linear wave amplitude envelope82D T Roper,S Sharma,R Sutton,and P CulverhouseProc.IMechE Vol.225Part M:J.Engineering for the Maritime Environmentproperties,similar to those reportedly used in real fish to increase metabolic efficiency while swimming [30].A study carried out by Harper et al .[31]sug-gested that energy savings of up to 30per cent could be achieved by using harmonically tuned springs to recapture inertial energy;however,no such savings have as yet been reported in practice.RoboPike’s forward section was constructed as a single pressure vessel housing batteries and elec-tronic subsystems;however,the tail and the mid-body were flooded.Without parameter optimization,RoboPike had a maximum speed of around 0.3L/s at a tail beat frequency of 1Hz.The Japanese National Maritime Research Institute (NMRI)developed a series of further sim-plified link-based robotic fish,including a three-link 34cm robotic sea bream denoted PF-300,which was built to study the turning performance [32].The sea bream was selected as a source of bioin-spiration because in nature its large side profile area and carangiform swimming style makes it an excellent fast-turning fish.The two joints were actu-ated directly by brushless d.c.servomotors housed in small pressure vessels;the actuation mechanism penetrated the pressure vessel through a corrugated waterproof boot [33].The tail itself was left in a naked skeletal state;as it was thought that the majority of the propulsive force would be generated by the caudal fin,accurate representation of the rest of the body morphology was thought to be unnecessary.Servo control came from a standard radio control unit.A float held the aerial at the surface and ensured that PF-300maintained a constant depth.PF-300was able to produce tail beat frequencies of up to 2.3Hz,turning diameters as small as 75mm could be achieved,and the top speed was approxi-mately 0.6L/s.Subsequent robot swimmers developed by NMRI,include the 65cm four-link PF-600,the 70cm four-link PF-700,the 97cm three-link UPF-2001,the 26cm two-link PF-200,and the 57cm three-link PF-550[33].PF-600,which was designed to study propulsion performance,had a cylindrical body housing two brushless d.c.servomotors;one servomotor actively controlled the two foremost joints,while the second servomotor was devoted entirely to actuating the caudal fin,allowing experimentation with phase angle between fin and tail movements.PF-700was built for experiments on fast swim-ming;the body had a long slim cylindrical form designed for low drag.Through the use of a combi-nation of a brushless d.c.servomotor and a larger d.c.motor driving a Scotch yolk mechanism,tail beat frequencies of up to 10Hz could be achieved,resulting in a top speed of 1L/s.UPF-2001was a simple three-link robot designed as a multi-purpose research platform.The tail was driven by a single d.c.motor and Scotch yoke mechanism,driving both the tail joint and the fin joint with a phase difference generated through a novel mechanical mechanism.Like the PF-700,a tail beat frequency of 10Hz was required to gener-ate a speed of 1L/s.PF-200was a small proof-of-concept prototype that used a shifting mass mechanism to give active pitch control for manoeuvring on the vertical axis.The most recent robotic swimmer from NMRI is PF-550,using a simple three-link design;actuation comes from two brushless d.c.servomotors,and the entire tail mechanism is mounted on arotatingFig.3A sectional view of Draper Laboratories’VCUUV (reproduced with permission of OxfordUniversity Press from Anderson and Chhabra [27])Developments towards biologically inspired propulsion systems for AUVs 83Proc.IMechE Vol.225Part M:J.Engineering for the Maritime Environmentshaft,allowing the primary propulsor to be rotated to give agility on the vertical axis.Like PF-300,all the subsequent robot swimmers to emerge from NMRI relied on radio communica-tion remote control,limiting them to operations on or near the surface.With the exception of UPF-2001 all were constructed with open skeletal joints;how-ever,effort was made to approximate the profile of real fish tails by attaching moulded sections to the tail vertebra.The Tokyo Institute of Technology developed two robotic dolphins aimed as prototypes toward the design of a biomimetically propelled AUV[34]. The first had a pneumatic actuation system,and the second a d.c.servomotor.Both robots had a three-vertebrae design1.5m in length with one active joint at the top of the tail, and a passive joint at the caudal fin.By varying the stiffness of the passive joint,it was found that a wide variety of tail beat kinematics could be achieved[35].The bodies were constructed from an aluminium frame wrapped in carbon-fibre-reinforced plastic.The deformable tail shapes were made from fibre-reinforced plastic rings connected by a non-elastic waterproof membrane.The stiff-ness of the passive joint was adjusted by inter-changing springs,of various elastic moduli. Through experimentation,it was found that speeds of around0.6L/s could be achieved with tail beat frequencies of around1.8Hz.The popularity of Gray’s paradox[20]makes dol-phins an obvious source of bioinspiration.So much work has been done investigating the paradox[21] that the swimming kinematics of dolphins are among the best understood in nature.Furthermore, their size in nature is comparable with the size of existing AUVs.Developers at the Istanbul Technical University also developed a robotic dolphin AUV prototype with the hope of improving upon the propulsion efficiency found in conventional AUVs[36].The Istanbul dolphin had a four-vertebrae construction with each of the three joints actuated by an oppos-ing bellows-type pneumatic system.The Istanbul dolphin was constructed from sheet aluminium and polymerized formaldehyde plastic.The flexing tail section was covered in a waterproof membrane supported by a flexible structure to allow the tail joints to remain dry.The caudal fin was made from cast silicon in order to mimic the flexibility of a real dolphin’s caudal fin.Turning mano-euvrability was achieved using pectoral fins actuat-ed in pitch.The Istanbul dolphin was able to swim at a speed of1L/s,with a tail beat frequency of 1.35Hz.In contrast,the University of Essex has developed a series of multi-link carangiform and subcarangi-form robot swimmers[37],the latest of which, namely G9,is based on a four-vertebrae tail struc-ture constructed using stereolithography apparatus resin.The three joints are actively controlled by three powerful d.c.servomotors,capable of bending the body through an angle of90°in0.2s[38].With G9,manoeuvres on the vertical axis are achieved through a shifting mass mechanism that moves the centre of gravity to alter the pitching moment, much like the mechanism in PF-200.No specific fish was chosen as a source of bioinspiration; instead an attempt was made to capture the more generalized principles of fish morphology.The debut of the Essex fish at the London Aquarium in2005has made it perhaps the most well-known robotic fish.They are currently being implemented in a collaborative project entitled ‘Search and monitoring of harmful contaminants, other pollutants and leaks in vessels in port using a swarm of robotic fish(SHOAL)’.The Beihang University Robotics Institute also developed a series of robotic fish based on non-specific bioinspired morphology for use as UUVs. SPC-II and SPC-III had a common two-joint BCF-type propulsion module.The two joints were each actuated by a150W brushless d.c.motor,located within a sealed part of the vehicle.In water the d.c. servomotors were capable of driving the tail with beat frequencies of up to2.5Hz.SPC-II had a roughly fish-like morphology designed like PF-300with a large side profile area for rapid turning ability,with an overall length of 1.2m.The forward part of the body was constructed as a rigid pressure vessel,and the tail mechanism was attached behind.The tail mechanism was capa-ble of driving SPC-II at speeds of up to1.2L/s and producing yaw rates of up to70°/s.Despite having a maximum depth rating of only5m the SPC-II proved useful as a visual assistant for underwater archaeology[39].SPC-III was constructed in many ways like a tradi-tional AUV;however,in place of the propeller the two-joint BCF tail was attached,as shown in Fig.4. With a1.6m rigid body section,the hydrodynamic shape enabled the propulsion system to drive the vessel at speeds of up to1.17L/s[40].Following on from a study that demonstrated that dead fish exposed to a harmonic stimulus could produce a forward-swimming gait[41], researchers at MIT have developed a simplified compliant body method for generating BCF swim-ming gaits in small biomimetic AUVs suitable for multi-agent survey tasks[42].84D T Roper,S Sharma,R Sutton,and P Culverhouse Proc.IMechE Vol.225Part M:J.Engineering for the Maritime Environment。
轮腿式爬楼梯机器人的机械系统设计
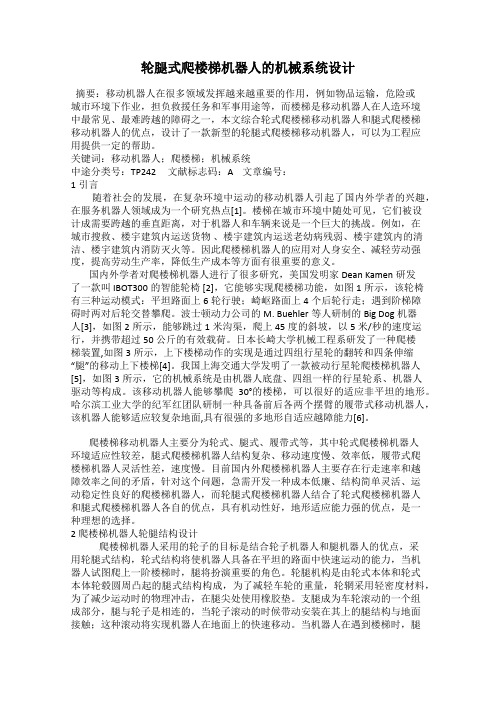
轮腿式爬楼梯机器人的机械系统设计摘要:移动机器人在很多领域发挥越来越重要的作用,例如物品运输,危险或城市环境下作业,担负救援任务和军事用途等,而楼梯是移动机器人在人造环境中最常见、最难跨越的障碍之一,本文综合轮式爬楼梯移动机器人和腿式爬楼梯移动机器人的优点,设计了一款新型的轮腿式爬楼梯移动机器人,可以为工程应用提供一定的帮助。
关键词:移动机器人;爬楼梯;机械系统中途分类号:TP242 文献标志码:A 文章编号:1引言随着社会的发展,在复杂环境中运动的移动机器人引起了国内外学者的兴趣,在服务机器人领域成为一个研究热点[1]。
楼梯在城市环境中随处可见,它们被设计成需要跨越的垂直距离,对于机器人和车辆来说是一个巨大的挑战。
例如,在城市搜救、楼宇建筑内运送货物、楼宇建筑内运送老幼病残弱、楼宇建筑内的清洁、楼宇建筑内消防灭火等。
因此爬楼梯机器人的应用对人身安全、减轻劳动强度,提高劳动生产率,降低生产成本等方面有很重要的意义。
国内外学者对爬楼梯机器人进行了很多研究,美国发明家Dean Kamen研发了一款叫 IBOT300的智能轮椅 [2],它能够实现爬楼梯功能,如图1所示,该轮椅有三种运动模式:平坦路面上6轮行驶;崎岖路面上4个后轮行走;遇到阶梯障碍时两对后轮交替攀爬。
波士顿动力公司的M. Buehler等人研制的Big Dog机器人[3],如图2所示,能够跳过1米沟渠,爬上45度的斜坡,以5米/秒的速度运行,并携带超过50公斤的有效载荷。
日本长崎大学机械工程系研发了一种爬楼梯装置,如图3所示,上下楼梯动作的实现是通过四组行星轮的翻转和四条伸缩“腿”的移动上下楼梯[4]。
我国上海交通大学发明了一款被动行星轮爬楼梯机器人[5],如图3所示,它的机械系统是由机器人底盘、四组一样的行星轮系、机器人驱动等构成。
该移动机器人能够攀爬30°的楼梯,可以很好的适应非平坦的地形。
哈尔滨工业大学的纪军红团队研制一种具备前后各两个摆臂的履带式移动机器人,该机器人能够适应较复杂地面,具有很强的多地形自适应越障能力[6]。
Advances in Material Science

Advances in Material ScienceMaterials science is a constantly evolving field that deals with the study of matter and materials, their properties, and how they can be shaped, engineered or applied for various purposes. From the discovery of new materials to the development of cutting-edge technologies, advances in material science have revolutionized the world around us. In this article, we will take a closer look at some of the recent breakthroughs and innovations that have been achieved in the field of material science.Nanomaterials and NanotechnologyOne of the most exciting developments in materials science is the emergence of nanotechnology. Nanotechnology deals with the manipulation of matter at the atomic, molecular, and supramolecular scale, and has opened up new possibilities for the creation of advanced materials with unique properties. Nanomaterials have a larger surface area to volume ratio than bulk materials, which gives them enhanced mechanical, optical, and chemical characteristics. Some of the most commonly used nanomaterials include graphene, carbon nanotubes, and quantum dots.One of the main applications of nanotechnology is in the field of electronics, where nanomaterials are used to create faster and more efficient components. Graphene, for example, is an ideal material for creating faster and more energy-efficient transistors, while carbon nanotubes are used to create high-end displays and sensors. In addition to electronics, nanotechnology is being used in the development of new drug delivery systems, advanced sensors, and more efficient batteries.Bio-inspired materialsNature is an endless source of inspiration for materials scientists, and many of the most innovative materials have been inspired by biology. Bio-inspired materials are designed to mimic natural systems and structures, and can have a range of applications in fields such as medicine, energy, and materials science. One example of a bio-inspiredmaterial is spider silk, which has excellent mechanical properties, is biodegradable, and can be produced in large quantities.Scientists are also using biologically inspired techniques to create self-healing materials. These are materials that can repair themselves when they are damaged, similar to how our skin heals after a cut. Self-healing materials have a range of potential applications, including in the automotive and aerospace industries, where they could help to reduce costs and increase longevity.3D Printing3D printing is a rapidly evolving technology that allows materials to be printed, layer by layer, to create complex structures. This technology has revolutionized the way that many industries create products, and has opened up new possibilities for materials science. 3D printing enables the creation of materials with unique shapes and properties that cannot be produced using traditional manufacturing techniques, and has led to the creation of stronger, lighter, and more resilient materials.One example of the use of 3D printing in materials science is in the development of new alloys. Multi-material alloys can be printed using 3D printing, which can combine the best properties of different metals to create new materials with enhanced characteristics. This technology is also being used to create customized medical implants and prosthetics, which can be tailored to the individual needs of each patient.Smart MaterialsSmart materials are materials that can respond to external stimuli, such as temperature, light, and pressure, and change their properties accordingly. These materials have a range of potential applications in engineering, aerospace, and medicine. For example, smart materials can be used in the aerospace industry to create self-healing surfaces on aircraft, or in the medical field to create drug delivery systems that release medications at specific intervals.Shape-memory alloys are one example of a smart material that has been particularly well-received. These alloys can change shape when exposed to heat or pressure, and canthen return to their original shape when cooled. Shape-memory alloys have applications in a range of fields, including medical implants, aerospace engineering, and smart textiles.ConclusionThe field of material science is constantly evolving, and the recent breakthroughs and innovations highlighted in this article are just the tip of the iceberg. From nanotechnology to smart materials, the possibilities for new materials and applications are limitless. These advances in material science have the potential to revolutionize various industries and improve our quality of life, and it is exciting to see what breakthroughs the future holds.。
英语作文从自然中获取灵感的发明
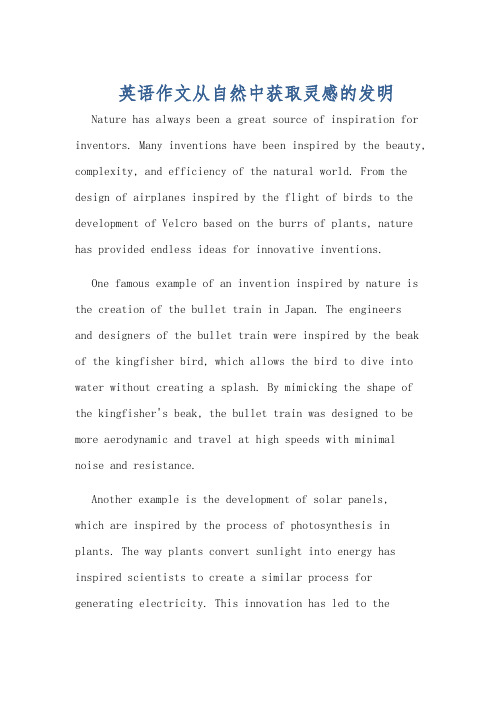
英语作文从自然中获取灵感的发明Nature has always been a great source of inspiration for inventors. Many inventions have been inspired by the beauty, complexity, and efficiency of the natural world. From the design of airplanes inspired by the flight of birds to the development of Velcro based on the burrs of plants, nature has provided endless ideas for innovative inventions.One famous example of an invention inspired by nature is the creation of the bullet train in Japan. The engineersand designers of the bullet train were inspired by the beak of the kingfisher bird, which allows the bird to dive into water without creating a splash. By mimicking the shape of the kingfisher's beak, the bullet train was designed to be more aerodynamic and travel at high speeds with minimal noise and resistance.Another example is the development of solar panels,which are inspired by the process of photosynthesis in plants. The way plants convert sunlight into energy has inspired scientists to create a similar process for generating electricity. This innovation has led to thewidespread use of solar panels as a clean and sustainable energy source.In addition, the study of sharkskin has led to the creation of a special type of paint that reduces drag on ships and improves fuel efficiency. By studying the unique texture of sharkskin, scientists were able to develop a paint that mimics its properties, leading to significant advancements in the field of marine transportation.These examples demonstrate how nature has inspired inventions that have improved our lives and the way we interact with the world. By observing and studying the natural world, inventors and scientists continue to find new ways to solve complex problems and create innovative technologies.自然一直是发明家们的灵感之源。
仿生学和机器人英语作文
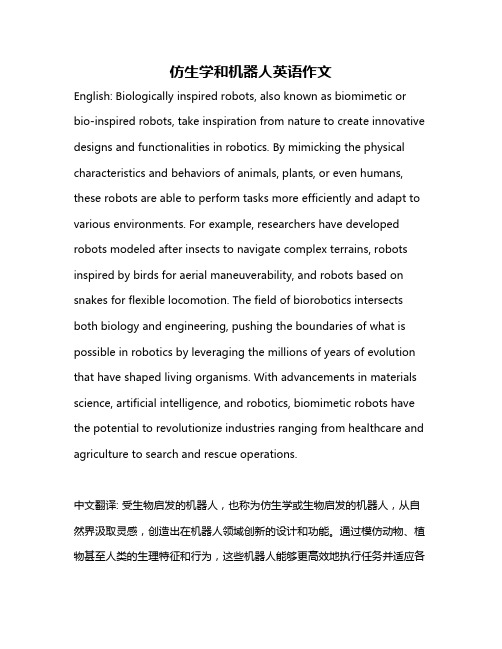
仿生学和机器人英语作文English: Biologically inspired robots, also known as biomimetic or bio-inspired robots, take inspiration from nature to create innovative designs and functionalities in robotics. By mimicking the physical characteristics and behaviors of animals, plants, or even humans, these robots are able to perform tasks more efficiently and adapt to various environments. For example, researchers have developed robots modeled after insects to navigate complex terrains, robots inspired by birds for aerial maneuverability, and robots based on snakes for flexible locomotion. The field of biorobotics intersects both biology and engineering, pushing the boundaries of what is possible in robotics by leveraging the millions of years of evolution that have shaped living organisms. With advancements in materials science, artificial intelligence, and robotics, biomimetic robots have the potential to revolutionize industries ranging from healthcare and agriculture to search and rescue operations.中文翻译: 受生物启发的机器人,也称为仿生学或生物启发的机器人,从自然界汲取灵感,创造出在机器人领域创新的设计和功能。
发明灵感源于自然英文作文

发明灵感源于自然英文作文Possible essay:Drawing Inspiration from Nature。
Nature has always been a source of wonder and inspiration for human beings. From the earliest cave paintings to the latest scientific discoveries, we have sought to understand and harness the power and beauty of the natural world. Many of the greatest inventions and innovations in human history have been inspired by nature, from the wheel to the airplane, from the telescope to the internet. In this essay, I will explore some examples of how inventors have drawn inspiration from nature and explain why this approach is still relevant and valuable today.One of the most famous examples of biomimicry, or the imitation of biological systems and processes, is the design of the airplane by the Wright brothers. Theyobserved how birds use their wings to generate lift and control their flight, and applied this principle to their own flying machine. They also studied the shape and structure of bird feathers and adapted them to their propellers and wings. By learning from nature, the Wright brothers were able to overcome the limitations of human-powered flight and achieve sustained, controlled flight for the first time in history.Another example of biomimicry is the invention of Velcro by Swiss engineer George de Mestral. He was inspired by the way burrs stick to his dog's fur during a walk in the woods and wondered how he could create a fastener that could be easily opened and closed like a burr. He examined the microscopic hooks and loops on the surface of the burr and developed a synthetic version that became the basis for Velcro. This simple yet versatile invention has since been used in countless applications, from clothing to aerospace.A more recent example of biomimicry is the development of self-healing materials by researchers at the University of Illinois. They were inspired by the way living organismsrepair themselves after injury or damage and sought to create synthetic materials that could do the same. They designed a polymer that could react to heat or light by forming new bonds and restoring its original properties. This breakthrough could have many applications in fields such as medicine, construction, and transportation.Why is drawing inspiration from nature still relevant and valuable today? One reason is that nature has evolved over millions of years to optimize efficiency, resilience, and adaptability. By studying and imitating these natural systems and processes, we can create more sustainable and effective solutions to our own problems. For example, by learning how plants capture and store solar energy through photosynthesis, we can develop better solar cells and batteries. By studying how animals move and navigate in complex environments, we can design more agile and autonomous robots. By mimicking the way ecosystems balance diversity and cooperation, we can improve our own social and economic systems.Another reason is that nature can inspire us toappreciate and protect the natural world. By recognizingthe beauty and complexity of nature, we can cultivate a sense of wonder and respect for the environment. Bylearning how nature provides us with essential resourcesand services, we can value and conserve them. By understanding how human activities affect the balance and health of ecosystems, we can take responsibility for our impact and seek to mitigate it.In conclusion, drawing inspiration from nature has been a timeless and fruitful approach to innovation and invention. By observing and learning from the natural world, we can create more efficient, sustainable, and resilient solutions to our own challenges. By appreciating and protecting nature, we can ensure a healthier and more harmonious relationship between humans and the environment. As Albert Einstein once said, "Look deep into nature, and then you will understand everything better."。
仿生学车草发明英语作文400字
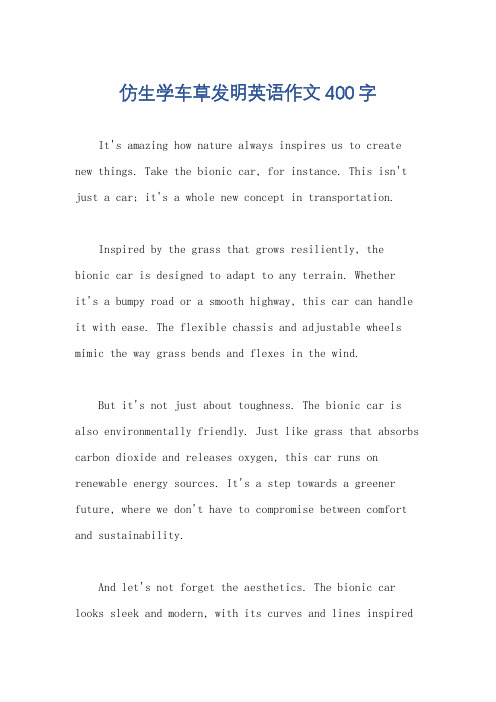
仿生学车草发明英语作文400字It's amazing how nature always inspires us to create new things. Take the bionic car, for instance. This isn't just a car; it's a whole new concept in transportation.Inspired by the grass that grows resiliently, the bionic car is designed to adapt to any terrain. Whetherit's a bumpy road or a smooth highway, this car can handle it with ease. The flexible chassis and adjustable wheels mimic the way grass bends and flexes in the wind.But it's not just about toughness. The bionic car is also environmentally friendly. Just like grass that absorbs carbon dioxide and releases oxygen, this car runs on renewable energy sources. It's a step towards a greener future, where we don't have to compromise between comfort and sustainability.And let's not forget the aesthetics. The bionic car looks sleek and modern, with its curves and lines inspiredby nature's own designs. Driving this car is like being one with nature, connected to the world in a whole new way.So in a nutshell, the bionic car is a testament to the power of nature and human ingenuity. It's a perfect blend of form and function, a true masterpiece of engineering.。
仿生设计发明英语作文
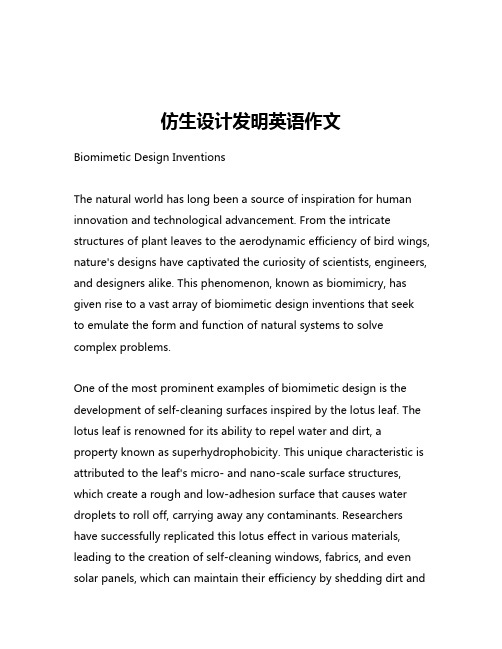
仿生设计发明英语作文Biomimetic Design InventionsThe natural world has long been a source of inspiration for human innovation and technological advancement. From the intricate structures of plant leaves to the aerodynamic efficiency of bird wings, nature's designs have captivated the curiosity of scientists, engineers, and designers alike. This phenomenon, known as biomimicry, has given rise to a vast array of biomimetic design inventions that seekto emulate the form and function of natural systems to solve complex problems.One of the most prominent examples of biomimetic design is the development of self-cleaning surfaces inspired by the lotus leaf. The lotus leaf is renowned for its ability to repel water and dirt, a property known as superhydrophobicity. This unique characteristic is attributed to the leaf's micro- and nano-scale surface structures, which create a rough and low-adhesion surface that causes water droplets to roll off, carrying away any contaminants. Researchers have successfully replicated this lotus effect in various materials, leading to the creation of self-cleaning windows, fabrics, and even solar panels, which can maintain their efficiency by shedding dirt anddebris.Another example of biomimetic design is the development of adhesive materials inspired by the remarkable sticking power of gecko feet. Geckos are able to effortlessly climb up smooth vertical surfaces and even hang upside down due to the millions of microscopic hair-like structures on their toes, known as setae. These setae create a strong van der Waals force, allowing geckos to adhere to a wide range of surfaces. Biomimetic researchers have developed synthetic adhesives that mimic the structure and function of gecko setae, leading to the creation of reusable and reversible adhesives that can be used in a variety of applications, from robotics to medical devices.The field of biomimetic design has also yielded innovative solutions in the realm of energy generation and storage. The unique structure of the Namibian desert beetle's back, which is able to condense water from the air, has inspired the development of water harvesting devices that can provide clean drinking water in arid regions. Similarly, the intricate patterns found in the wings of certain butterfly species have been replicated in the design of solar cells, leading to increased light-harvesting efficiency and the potential for more compact and lightweight solar panels.In the field of robotics, biomimicry has been instrumental in thedevelopment of highly maneuverable and energy-efficient machines. The graceful and efficient movements of animals such as cheetahs, octopuses, and insects have been studied and replicated in the design of robotic limbs and locomotion systems. These biomimetic robots can navigate complex environments, perform delicate tasks, and even mimic the camouflage abilities of certain species, opening up new possibilities for applications in fields ranging from search and rescue operations to environmental monitoring.Beyond these well-known examples, the influence of biomimicry can be seen in a wide range of other inventions and technologies. Researchers have drawn inspiration from the self-healing properties of certain plants to develop advanced materials that can repair themselves, while the aerodynamic principles observed in the wings of birds and insects have been applied to the design of more fuel-efficient aircraft and wind turbines.As our understanding of the natural world continues to deepen, the potential for biomimetic design to drive innovation and solve pressing global challenges only continues to grow. By studying and emulating the elegant solutions evolved by nature over millions of years, we can unlock new avenues for technological progress and create a more sustainable future. The biomimetic design inventions of today are just the beginning of a new era of human-naturecollaboration, where the wisdom of the natural world guides us towards a more harmonious and innovative future.。
仿生学前车草英语作文300字左右
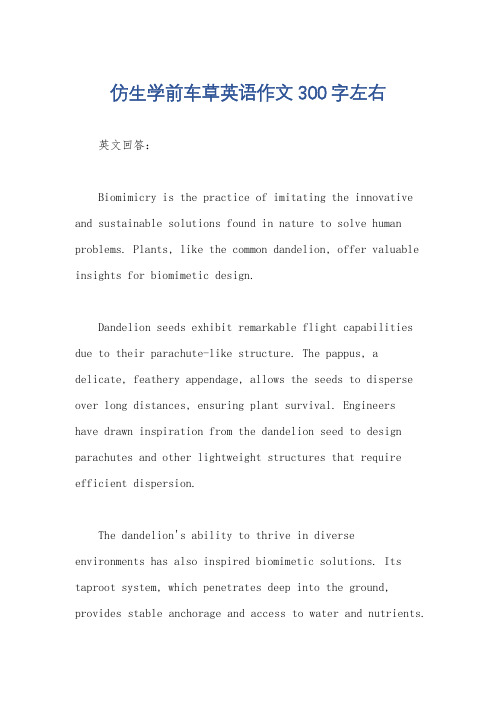
仿生学前车草英语作文300字左右英文回答:Biomimicry is the practice of imitating the innovative and sustainable solutions found in nature to solve human problems. Plants, like the common dandelion, offer valuable insights for biomimetic design.Dandelion seeds exhibit remarkable flight capabilities due to their parachute-like structure. The pappus, a delicate, feathery appendage, allows the seeds to disperse over long distances, ensuring plant survival. Engineershave drawn inspiration from the dandelion seed to design parachutes and other lightweight structures that require efficient dispersion.The dandelion's ability to thrive in diverse environments has also inspired biomimetic solutions. Its taproot system, which penetrates deep into the ground, provides stable anchorage and access to water and nutrients.This design principle has been applied in the developmentof lightweight and efficient anchoring systems for construction and engineering applications.Moreover, the dandelion's resilience to environmental stressors has attracted scientific interest. Its waxyleaves repel water, preventing dehydration and disease. Scientists are exploring biomimetic approaches to create waterproof materials and surfaces inspired by thedandelion's leaf structure.中文回答:仿生学是模仿自然界中创新的可持续解决方案来解决人类问题的实践。
汽车模型设计理念文案英文
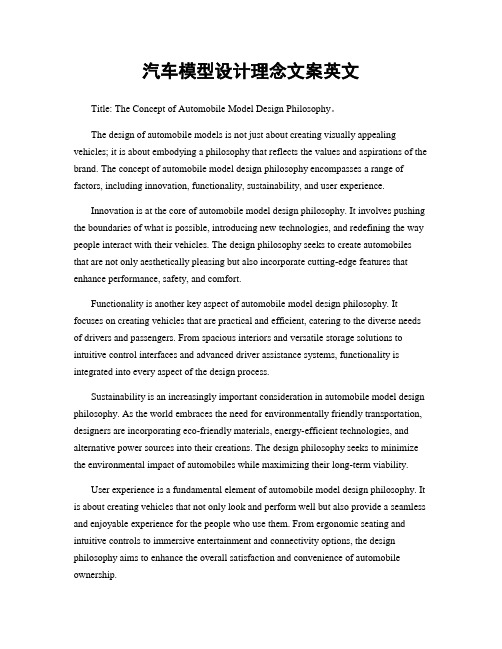
汽车模型设计理念文案英文Title: The Concept of Automobile Model Design Philosophy。
The design of automobile models is not just about creating visually appealing vehicles; it is about embodying a philosophy that reflects the values and aspirations of the brand. The concept of automobile model design philosophy encompasses a range of factors, including innovation, functionality, sustainability, and user experience.Innovation is at the core of automobile model design philosophy. It involves pushing the boundaries of what is possible, introducing new technologies, and redefining the way people interact with their vehicles. The design philosophy seeks to create automobiles that are not only aesthetically pleasing but also incorporate cutting-edge features that enhance performance, safety, and comfort.Functionality is another key aspect of automobile model design philosophy. It focuses on creating vehicles that are practical and efficient, catering to the diverse needs of drivers and passengers. From spacious interiors and versatile storage solutions to intuitive control interfaces and advanced driver assistance systems, functionality is integrated into every aspect of the design process.Sustainability is an increasingly important consideration in automobile model design philosophy. As the world embraces the need for environmentally friendly transportation, designers are incorporating eco-friendly materials, energy-efficient technologies, and alternative power sources into their creations. The design philosophy seeks to minimize the environmental impact of automobiles while maximizing their long-term viability.User experience is a fundamental element of automobile model design philosophy. It is about creating vehicles that not only look and perform well but also provide a seamless and enjoyable experience for the people who use them. From ergonomic seating and intuitive controls to immersive entertainment and connectivity options, the design philosophy aims to enhance the overall satisfaction and convenience of automobile ownership.In conclusion, the concept of automobile model design philosophy is a multifaceted approach that encompasses innovation, functionality, sustainability, and user experience. It is about creating vehicles that not only reflect the values and aspirations of the brand but also meet the evolving needs and expectations of drivers and passengers. By embracing this design philosophy, automobile manufacturers can create vehicles that are not only visually striking but also practical, sustainable, and enjoyable to use.。
beyond the closed sensorimotor
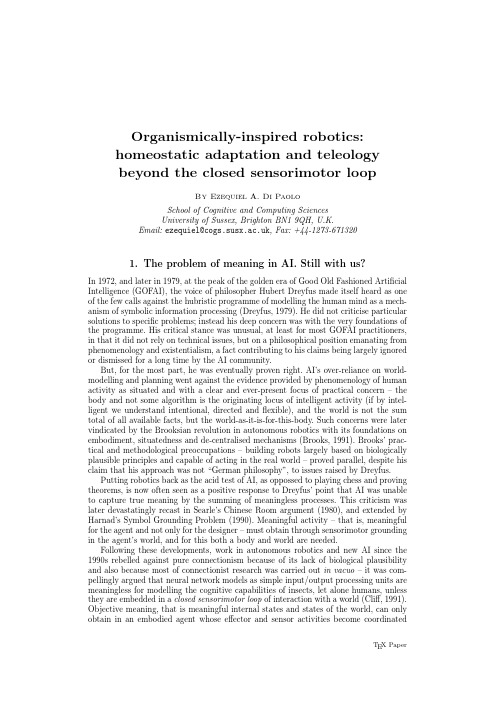
Organismically-inspired robotics: homeostatic adaptation and teleologybeyond the closed sensorimotor loopBy Ezequiel A.Di PaoloSchool of Cognitive and Computing SciencesUniversity of Sussex,Brighton BN19QH,U.K.Email:ezequiel@,Fax:+44-1273-6713201.The problem of meaning in AI.Still with us?In1972,and later in1979,at the peak of the golden era of Good Old Fashioned Artificial Intelligence(GOFAI),the voice of philosopher Hubert Dreyfus made itself heard as one of the few calls against the hubristic programme of modelling the human mind as a mech-anism of symbolic information processing(Dreyfus,1979).He did not criticise particular solutions to specific problems;instead his deep concern was with the very foundations of the programme.His critical stance was unusual,at least for most GOFAI practitioners, in that it did not rely on technical issues,but on a philosophical position emanating from phenomenology and existentialism,a fact contributing to his claims being largely ignored or dismissed for a long time by the AI community.But,for the most part,he was eventually proven right.AI’s over-reliance on world-modelling and planning went against the evidence provided by phenomenology of human activity as situated and with a clear and ever-present focus of practical concern–the body and not some algorithm is the originating locus of intelligent activity(if by intel-ligent we understand intentional,directed andflexible),and the world is not the sum total of all available facts,but the world-as-it-is-for-this-body.Such concerns were later vindicated by the Brooksian revolution in autonomous robotics with its foundations on embodiment,situatedness and de-centralised mechanisms(Brooks,1991).Brooks’prac-tical and methodological preoccupations–building robots largely based on biologically plausible principles and capable of acting in the real world–proved parallel,despite his claim that his approach was not“German philosophy”,to issues raised by Dreyfus.Putting robotics back as the acid test of AI,as oppossed to playing chess and proving theorems,is now often seen as a positive response to Dreyfus’point that AI was unable to capture true meaning by the summing of meaningless processes.This criticism was later devastatingly recast in Searle’s Chinese Room argument(1980),and extended by Harnad’s Symbol Grounding Problem(1990).Meaningful activity–that is,meaningful for the agent and not only for the designer–must obtain through sensorimotor grounding in the agent’s world,and for this both a body and world are needed.Following these developments,work in autonomous robotics and new AI since the 1990s rebelled against pure connectionism because of its lack of biological plausibility and also because most of connectionist research was carried out in vacuo–it was com-pellingly argued that neural network models as simple input/output processing units are meaningless for modelling the cognitive capabilities of insects,let alone humans,unless they are embedded in a closed sensorimotor loop of interaction with a world(Cliff,1991). Objective meaning,that is meaningful internal states and states of the world,can only obtain in an embodied agent whose effector and sensor activities become coordinatedT E X Paper2 E.A.Di Paolowhilst performing the desired task.Neural network models of cognition can of course be abstract and simplified,but simplifying the sensorimotor loop out of which the contin-gencies and invariants necessary for stable perception and behaviour originate,we now believe,is akin to modelling supersonicflight and ignoring gravity.Researchers working in new approaches to AI today(Pfeifer&Scheier,1999;Harvey et al.,1997;Nolfi&Floreano,2000;Brooks et al.,1999;Beer,2000)are pretty well aware of these events that shaped the recent history of theirfield.Many of the problems outlined by Dreyfus became issues of(often practical)concern that had to be resolved typically with a turn towards aspects of real instances of cognition,what Varela called the re-enchantment of the concrete(Varela,1995).This turn often became more compelling as we started to look at animal behaviour more closely.The exceptions provided by human intelligence,its ability for detachment and generality,have often been a stumbling block in the way to understanding the embodied and situated nature of cognition.The turn towards the concrete,in robotics,is mainly a turn towards the biological.And biological inspiration is now one of the proudest labels for the new robotics.My purpose in this paper is to ask whether the slow unravelling of this story,the re-discovery through different avenues of what Dreyfus saw as the fundamental problems of AI already in the1970s and which often has implied turning back to insights generated before the GOFAI age in cybernetics and holistic approaches to biology and psychology, has reached an end or whether something fundamental is still missing.I will argue for the second option.I think we have not yet seen the ending of this story,but that all the elements are in place at this very moment for moving on to the next chapter.As before,practical concerns will be strong driving motivations for the development of the necessary ideas.I will try to raise those concerns with the humble aim of putting them on the discussion table.I will not claim to solve anything although I will hint at possible solutions and discuss how good they seem to be at this stage.In a nutshell,my claim is that autonomous robots still lack one fundamental property of what makes real animals cognitive agents.A property that motivated Dreyfus to argue against GOFAI.This property is that of intentional agency.Contemporary robots,I will argue,cannot be rightly seen as centres of concern,or put simply as subjects,the way that animals can.A robot failing in its performance does not show any signs of preoccupation –failure or success do not affect its structure in any dangerous way,nor does any form of concern accompany its actions simply because the desired goal is not desired by the robot but by the designer.Such robots can never be truly autonomous.In other words the presence of a closed sensorimotor loop does not fully solve the problem of meaning in AI.And this,as we shall see,is not merely a complexity issue(such is currently the widespread belief in the robotics community)but,as before,a question of not attempting to modelflight without including gravity.The problem,it is clear,will be to pin down such a general criticism to a concrete form,so that we can glimpse some technical response to the challenge.For this,I must be able to clarify what could one possibly mean with a statement such as“a robot has no intentions”.I propose to do this by looking at the relation between intrinsic teleology and life as exposed in the philosophy of Hans Jonas(who is in fact representative of a larger movement of mainly Continental approaches to the philosophy of biology) and the convergence of these ideas with theories of self-organisation and autopoiesis;a convergence that has been described very recently(Weber&Varela,2002).I will then ask the question of how close can we design a robot to not just to resemble but to be like an animal.This paper therefore falls within the class of recent efforts that argue for some sort of continuity between life and cognition(Maturana&Varela,1980;Stewart, 1996;Wheeler,1997).The answer to these questions is definitely not of the additive kind.It will not be a matter offinding the missing ingredient so that it can be included in our robot design in aOrganismically-inspired robotics3 box labelled Emotion or Motivation or Value System.Such attempts exist and generate results of interest which are perfectly valid in other contexts,such as modelling.But from the point of view of this discussion they miss the point,as I shall argue.The last part of this paper will deploy an alternative path based on Ashby’s(1960)framework for adaptive behaviour applied to the process of habit formation,finishing with recent work on homeostatic adaptation(Di Paolo,2000).Whether these solutions will prove useful is less important than raising awareness of the problem and generating debate.2.The problem:In what ways are robots different from animals? The label“biologically-inspired”has been applied to important advances in autonomous robotics,starting from Brooks’criticism of the sense-model-plan-act GOFAI approach to robot design as one that is unsupported by biological data(Brooks,1991),moving on to the exploration of mechanisms,both neural and bodily,directly inspired on neuroscien-tific,physiological and ethological data to the effect of making robots more autonomous, more adaptable and more animal-like.An extremely fruitful way of working in synthetic approaches to robotics is the more or less systematic exploration of biological mechanisms not typically included in robot design.There’s hardly a case where such an exploration, if done well,doesn’t yield some interesting result.In this respect it is possible to mention the work of Husbands and colleagues(Hus-bands et al.,1998)on GasNets,neural robot controllers where single nodes are capable of emitting and responding to diffusible neuromodulators,and the work of Floreano and Urzelai(2000)on the evolution of synaptic plasticity.Recent explorations have also turned to networks of spiking neurons(Floreano&Mattiussi,2001)and spike-timing dependent plasticity(Di Paolo,2003)by harnessing the power of evolutionary design to synthesize appropriate robot controllers which can later be the subject of analysis and may,eventually,feed information back to neuroscience by providing exemplars of whole-agent,closed-sensorimotor-loop control–something not typically explored in com-putational neuroscience.Biological inspiration has also influenced the design of robot body plans and sensorimotor arrays.Embodiment is often given a concrete,if perhaps sometimes limited,meaning by studying to what extent a body plan,a particular set of effectors or sensors,contributes towards the effectiveness of robot performance.Ex-amples abound from simple,but powerful proofs of concept(e.g.,Didabot,(Pfeifer& Scheier,1999)),to complex insect-like and snake-like structures and humanoid robots. Often these designs are not static,but make use of passive dynamics principles and loose couplings through both the environment and other bodily structures to achieve robust and adaptable performance.In this respect,we can mention the whole sub-discipline of passive-dynamic walking(McGeer,1990;Collins et al.,2001)and the work on joint control using bodily coupled neural oscillators(Williamson,1998).Further influences of a biologically-inspired frame of mind are sometimes subtler. Robot design is less seen as a task whereby a mechanism must control a body to achieve unconditional performance but,more often,the controller-body-niche coupling has be-come the object of design.A controller works with a specific body design as much as the body works with the controller.The robotic task is not expected to persist if the environ-mental coupling is changed radically,as much as an animal is not labelled as lacking in intelligence if its niche is fundamentally altered and its performance non-adaptive.This change of perspective is sometimes unspoken,a sort of growing consensus in how the aims of autonomous robotics should be approached.An excellent discussion and further review can be found in(Chiel&Beer,1997;Beer et al.,1998).Undoubtedly,there is still much to be done and discovered in this research approach to robotics.So,trying to point to its limitations could be seen at best as premature or, less charitably,simply as whining.I will try in the following to spell out in what important4 E.A.Di Paoloways biologically-inspired robotics is missing out on perhaps the most crucial aspect of living organisms,and what a radical change paying attention to this aspect could bring about.But in doing so,I am trying to add to what is currently being done,not to criticise it negatively,because the current approach has not yet reached a stage that needs such criticism.If it turned into a blind dogma,if it limited itself to merely copying just-another-unexplored-mechanism-found-in-animals,the same way that much of research in connectionism is limited to studying yet-another-variant-of-a-learning-algorithm,then perhaps a wake-up call would be necessary.So far,such has not been the case.My contention,sketched here and further developed in the next sections,is that robot design may be getting important inspiration from the properties of biological neuronal mechanisms and from the dynamics of animal bodies,but it is getting little or no in-spiration from the fact that the organisms that serve as models are living systems and that they structure their activities and their environment into a space of meaning which is defined as that which distinguishes between what is relevant and what is irrelevant to the organism’s continuing mode of existence and ultimately survival.The crucial as-pect that I would like to emphasise is that such Umwelt,in the words of von Uexk¨u ll (1934),is not divorced from the internal organisation of the organism.On the contrary, it is both generated by this organisation and causally connected to its conservation and subsistence,i.e.,to what defines this organisation as that of a living being.What an organism is and what it does are not properties external to each other that must be brought into coordination by some additional process(natural selection being the favourite candidate here).The organisation of a living system by itself creates an agency.By cleaving the material space into what the organism is and what is not,by forming such a concrete boundary,the living organisation generates and maintains a relation between the organism and its exterior.By being an organisation of continued material renovation,the relation generated with the outside is a relation of need and satisfaction.Need that originates in the thermodynamic constraints of a structure in constant material and energeticflow,satisfaction when those constraints are met.In the particular case of animals,by putting a distance and a lapsus between the tensions of need and the consummation of satisfaction,the living organisation gives origin to a special relation with the outside,that of perception and action,which are charged with internal significance,and hence with grounded emotion.It is because of this that external events have concrete meaning for an organism–a meaning that is directly linked to whether its modes of being will continue or cease.These statements need further unpacking,and I propose to do this in the next section, but we can already put some emphasis on the main issues:1)an animal is a natural agent who generates its own boundaries and defines its world,a robot is an agent in virtue of external conventions of spatiotemporal and material continuity;2)as a corollary,an animal does not simply have purposes,but generates them,a robot,alas,only has them, and it has them in a restricted sense solely in virtue of an externally imposed connection between its organisation and its environment.The relation of reciprocal causality that obtains in the animal,that between what it is and what it does and endures,appears in a broken version in the robot.Only the outward arm of the closed loop has occupied researchers:how to design a robot in order for it to do something.The way in which a robot’s behaviour and the perturbations it must face may challenge and shape its organisation,that is,the inward arm of the causal loop that is the necessary condition for a natural agency to arise,has not been an object of design in itself(although some recent work is starting to move in this direction,see below).Biological inspiration,at least up to this point,has thus been found to be of a limited scope–that of simulating and testing particular mechanisms found in animals.However, biologically-inspired robots are not necessarily organismically-inspired,i.e.,inspiration has not come from the defining organisation and conditions of the living,or some of itsOrganismically-inspired robotics5 corollaries.In the following,I will try to expand on the seriousness of this criticism.At this stage it may seem a bit convoluted,or even pretentious,to point out that robots are not like living systems,but it will be possible to show that taking this point seriously will have significant effects of a practical nature,in particular having to do with robotic autonomy, adaptivity and intentionality,as well as effects on research and design methodologies.3.Life,autonomy and identityBefore we formulate the question of whether it is possible to reproduce,at least in part,the fundamental organisation of living beings in an artefact,and what might the consequences of such an inclusion be,it is necessary to expend some effort in describing this organisation.This is a complex and rich subject which will by no means be exhausted in the next few paragraphs.I will only provide a sketch that will hopefully be complete enough for the purposes of the current discussion.The reader may wish to follow some of the references tofind more extensive and profound treatments of the subject.What is life?It is interesting how this question is more likely to be asked by a philosopher than a biologist.The historical reasons for this may turn out to be connected with the very answer to the question.Science seeks to explain phenomena in terms of underlying causal laws.If anything like circular causality orfinal causes are allowed, it is in the hygienized sense of“as-if”discourse.The term teleonomy(Nagel,1977)is meant to precisely describe such an use.In this sense,we may speak of an organism’s activities as directed towards an end,or of its morphology and physiology as serving a certain purpose,but in fact this is meant to capture regular correlations between an effect and its causes given a context of norms which is“tuned”–that is,brought into coordination–by some external process such as evolution and not internally generated by the organism itself.In this sense,an adaptive reaction in a predator anticipating a change in direction of thefleeing prey is something that has been acquired because it has been selected from a pool of random options as the one that better enhances the chances of survival.This etiological sense of functionality and purpose,see for instance (Millikan,1984),has little to do with the question we are trying to answer.This sense of teleonomy,of“as-if”functionality,is equally applicable to non-living artefacts,as long as they can be shown to have the right historical events shaping their current structures and that their structures can be spoken about as serving functions whose origin are those same historical events.Such is the kind of functionality that may be found,for instance, in evolutionary robotics.It makes sense also that we should apply a definition of life to situations where we know nothing about shaping historical events such as phylogeny.We should be able to recognize immediately other forms of life as living even though we may know nothing about their evolutionary history.In other words,a definition of life should be operational (Varela,1979),i.e.,based on what can be explained about a concrete instance without appealing to historical or contextual knowledge which by its very nature extends beyond the living system as it is given in front us into what has happened before of what may happen if we alter the current circumstances.It is clear,however,that a biology based on a Newtonian model will be comfortable with the above sense of teleonomy and that issues that may challenge this situation are less likely to be openly discussed by biologists;for instance,speaking of organisms as nat-ural and intrinsic purposes as Kant did in the second part of his Critique of Judgement. This is why philosophers,or philosophically minded scientists,have been more at ease with the question of life than many biologists.In the last century,an interesting conver-gence has occurred bringing together modern views of self-organisation and autopoiesis with existential and holistic takes on the question of life and behaviour.Scientists such as Plessner,Goldstein,Buytendijk,von Uexk¨u ll,Goodwin,Maturana,Varela,Rosen,and6 E.A.Di PaoloPattee have arrived,via diverse paths,to similar or close enough locations as philoso-phers such as Jonas,Merleau-Ponty and Straus.Such a convergence has been recently remarked by Weber and Varela(2002)in a discussion to which this paper owes much. The reader may also want to consult some of the main and secondary sources directly: (Maturana&Varela,1980;Varela,1979;Rosen,1991;Goldstein,1934;Goodwin&Web-ster,1997;Uexk¨u ll,1934;Straus,1966;Jonas,1966;Merleau-Ponty,1963;Grene,1968; Lenoir,1982;Harrington,1996).The most emblematic way of describing this convergence is in terms of an equal rejection of vitalism and other mystical and dualistic descriptions of life,and mechanicism,the Newtonian understanding of matter as inert and incapable of organisation unless this is provided by external forces.Hans Jonas(1966)has examined the nature of organisms from an existential point of view.He puts thefinger on metabolism as the distinguishing feature of life.A living organism does not posses the same kind of identity as a particle of matter.The latter is the‘same’entity in virtue of its spatiotemporal continuity.It is always‘this one’as opposed to‘that one’because of its material permanence.An organism,in contrast, stands out by the fact that it never actually coincides with its material constitution at a given instant.Due to its metabolism,its components are in constantflux.However, they maintain an organisation which assures its own durability in the face of randomising events that tend towards its dissolution.The organism has a formal and dynamic identity. It only coincides fully with its material constitution when it is dead.Machines can also be seen as having aflux of matter and energy,typically in the form of inputs and outputs but,Jonas argues,the organism is unlike any machine in that the very nature of theflux is used to fabricate and maintain its own components.These are not externally given,nor have they any independent identity.If metabolism stops,the organism ceases to be(or adopts a non-living form with the potential of re-starting life at a later stage,such as a seed).If a machine stops,it simply stops,it does not turn into something else.This can be re-stated as the fact that an organism’s identity is given by its own functioning,or that the organism self-produces.At this point we may notice the strong parallel between this point of view and the one presented by the theory of autopoiesis. This framework developed by Humberto Maturana and Francisco Varela(1980,1987) is based on the notion of autopoiesis,self-production,as the central property of living systems(as opposed to traditional“shopping list”definitions that merely state properties shared by many living systems).Accordingly,“An autopoietic system is organized(defined as unity)as a network of processes of production(synthesis and destruction)of components such thatthese components:(i)continuously regenerate and realize the network thatproduces them,and(ii)constitute the system as a distinguishable unity inthe domain in which they exist”,(Varela,1997).Formally stated,this embraces the definition proposed by Jonas and others such as Piaget (1967)back to Kant in the second part of the Critique of Judgement.The more existential aspects of Jonas’biophilosophy should not occupy us here,but are worth mentioning because they are surprising.Thefirst is that,admittedly,describing an organism in terms of its form,that is,as a centre of material and energeticflux, may after all be a matter of epistemological convenience.It is as describing a pattern of oscillating particles in a continuum as a wave.Once we know what each particle is doing,we know all about the wave,so the wave as an independent entity is just a useful, butfictitious,way of seeing the phenomenon of oscillating particles.Such,he argues, would be the way a mathematical mind would see life,as an epistemologically convenient embracing metaphor for particles that dance in a complex sort of wave.However,we can do better than that.We can ascertain beyond any shadow of a doubt that organismsOrganismically-inspired robotics7 have an identity beyond the epistemological convenience of detached description.In living systems“nature springs an ontological surprise in which the world-accident of terrestrial conditions brings to light an entirely new possibility of being:systems of matter that are unities of a manifold,not in virtue of a synthesizing perception whose object they happen to be,nor by the mere concurrence of the forces that bind their parts together,but in virtue of themselves,for the sake of themselves,and continually sustained by themselves”, (Jonas,1966,p.79).The way we know this for certain is simply that we are organisms. We know by the direct availability of our bodies and by our struggles that we are indeed one of these entities.We have,as Jonas puts it,inside knowledge–a knowledge that is not available to a disembodied and mathematical mind.This interesting existential turn in the argument,one that is difficult to argue with,is followed by an interesting corollary. If living systems are part of nature’s ontology,and if they constantly depend on matter at a given time,but are not attached to a specific collection of particles through time, then their relation to matter,and so to the laws that govern matter,is one of need on the one hand and freedom on the anisms are a wave of matter and energy,they are bound by the laws of physics but not fully determined by them as their destiny is not attached to any particular material configuration but they ride from one configuration to another.Jonas argues how this relation of needful freedom starts with metabolism but is later exploited and expanded by evolution in animals and eventually in humans.But this is beyond the scope of this paper.More relevant for our purposes–determining what use we can make of these ideas for designing organismically-inspired robots–is the following question:Why is the living organisation so special and difficult to describe?Because it refers at the same time to the processes of constitution of the organism,processes that define what the organism is,and to how those processes are generative of the organism as an agency,i.e.,what the organism does.The two are complementary aspects of the same entity.A typical systemic description starts with a system which is well-defined in terms of components and their relations.A living system is such only in virtue of the constant threat of becoming a different system or even a dead one.We may observe some stability in the organisation of an organism and be happy to constrain a model spatially to unchanging fragments of the organism or temporally by assuming that the organism will not change significantly in a given period.But we cannot truly model what is proper to the living organisation unless we leave room in our descriptions for the fact that this system could,at any given time,become something quite different.This threat,even if kept at bay by the adaptivity of the living organisation,is(negatively put)part of the defining property of life.We cannot understand life conceptually otherwise.Life,in short,is defined in terms of death and the threat of change.Allowing the possibility of ongoing shifts in the identity of the system in question, makes it quite difficult for scientists to model life(or closer to our concerns here,for a roboticist to create an artificial living robot).This is because all models must be grounded on a concrete description of the system being modelled.In other words,they rely on an identity of components based on continuity,and not on the self-generated identity of the organism.Living systems may change their structures as long as the autopoietic core is maintained.This means that the description of the system is never fixed but subject to constant ponents may come and go,and their relations change.Eventually even the autopoietic organisational core will be lost(when the system dies).This means that a successful model of a living system should involve a description of the system while the description itself is contingent to what the system does!Ultimately,when death arrives,the model should be not of a“system”at all, but of aggregates of inanimate components.Some people think such a modelling feat is impossible,I say that the very least is extremely difficult to conceive within the current modelling paradigms.Simulation modelling,with its potential for capturing different。
Bio-inspired Robotics Design
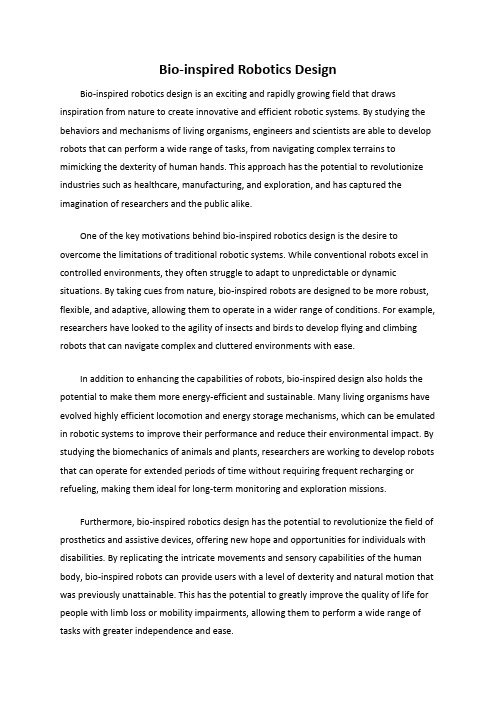
Bio-inspired Robotics DesignBio-inspired robotics design is an exciting and rapidly growing field that draws inspiration from nature to create innovative and efficient robotic systems. By studying the behaviors and mechanisms of living organisms, engineers and scientists are able to develop robots that can perform a wide range of tasks, from navigating complex terrains to mimicking the dexterity of human hands. This approach has the potential to revolutionize industries such as healthcare, manufacturing, and exploration, and has captured the imagination of researchers and the public alike.One of the key motivations behind bio-inspired robotics design is the desire to overcome the limitations of traditional robotic systems. While conventional robots excel in controlled environments, they often struggle to adapt to unpredictable or dynamic situations. By taking cues from nature, bio-inspired robots are designed to be more robust, flexible, and adaptive, allowing them to operate in a wider range of conditions. For example, researchers have looked to the agility of insects and birds to develop flying and climbing robots that can navigate complex and cluttered environments with ease.In addition to enhancing the capabilities of robots, bio-inspired design also holds the potential to make them more energy-efficient and sustainable. Many living organisms have evolved highly efficient locomotion and energy storage mechanisms, which can be emulated in robotic systems to improve their performance and reduce their environmental impact. By studying the biomechanics of animals and plants, researchers are working to develop robots that can operate for extended periods of time without requiring frequent recharging or refueling, making them ideal for long-term monitoring and exploration missions.Furthermore, bio-inspired robotics design has the potential to revolutionize the field of prosthetics and assistive devices, offering new hope and opportunities for individuals with disabilities. By replicating the intricate movements and sensory capabilities of the human body, bio-inspired robots can provide users with a level of dexterity and natural motion that was previously unattainable. This has the potential to greatly improve the quality of life for people with limb loss or mobility impairments, allowing them to perform a wide range of tasks with greater independence and ease.Despite the many exciting possibilities that bio-inspired robotics design presents, there are also significant challenges that must be overcome in order to fully realize its potential. One of the main obstacles is the complexity of biological systems, which often involve intricate interactions between multiple components at various scales. Replicating these systems in robotic form requires a deep understanding of biology, as well as advanced engineering techniques and materials. Additionally, there are ethical considerations to take into account, particularly when it comes to the use of animals as models for robotic design. It is important for researchers to approach this field with a strong sense of responsibility and respect for the natural world.In conclusion, bio-inspired robotics design is a fascinating and promising field that has the potential to transform the way we interact with technology. By drawing inspiration from the natural world, researchers are developing robots that are more capable, efficient, and versatile than ever before. From improving industrial processes to enhancing the lives of individuals with disabilities, the impact of bio-inspired robotics design is far-reaching and has the potential to bring about significant positive change. As this field continues to evolve, it will be important for researchers to collaborate across disciplines and maintain a strong ethical framework, ensuring that the benefits of bio-inspired robotics design are realized in a responsible and sustainable manner.。
六足机器人的发展史
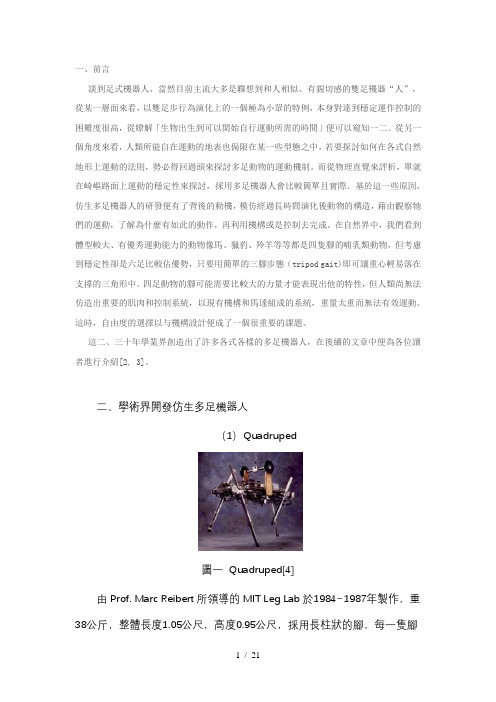
一、前言談到足式機器人,當然目前主流大多是聯想到和人相似、有親切感的雙足機器“人”,從某一層面來看,以雙足步行為演化上的一個極為小眾的特例,本身對達到穩定運作控制的困難度很高,從瞭解「生物出生到可以開始自行運動所需的時間」便可以窺知一二。
從另一個角度來看,人類所能自在運動的地表也侷限在某一些型態之中,若要探討如何在各式自然地形上運動的法則,勢必得回過頭來探討多足動物的運動機制。
而從物理直覺來評析,單就在崎嶇路面上運動的穩定性來探討,採用多足機器人會比較簡單且實際。
基於這一些原因,仿生多足機器人的研發便有了背後的動機,模仿經過長時間演化後動物的構造,藉由觀察牠們的運動,了解為什麼有如此的動作,再利用機構或是控制去完成。
在自然界中,我們看到體型較大、有優秀運動能力的動物像馬、獵豹、羚羊等等都是四隻腳的哺乳類動物,但考慮到穩定性卻是六足比較佔優勢,只要用簡單的三腳步態(tripod gait)即可讓重心輕易落在支撐的三角形中。
四足動物的腳可能需要比較大的力量才能表現出他的特性,但人類尚無法仿造出重要的肌肉和控制系統,以現有機構和馬達組成的系統,重量太重而無法有效運動。
這時,自由度的選擇以与機構設計便成了一個很重要的課題。
這二、三十年學業界創造出了許多各式各樣的多足機器人,在後續的文章中便為各位讀者進行介紹[2, 3]。
二、學術界開發仿生多足機器人(1)Quadruped圖一Quadruped[4]由Prof. Marc Reibert所領導的MIT Leg Lab於1984~1987年製作,重38公斤,整體長度1.05公尺,高度0.95公尺,採用長柱狀的腳,每一隻腳連接身體的關節是由兩個液壓致動器(hydraulic actuators)組成,分別控制腳的前後与左右的旋轉,腳上有一個線性致動器來提供推進力。
在控制上將腳簡易的分成兩組,不同的分組方法便產生了小跑(trot)、跑(pace)和奔馳(bound)等三種步態,奔馳可達到3.5個身體長度,有很好的穩定性和運動特性。
- 1、下载文档前请自行甄别文档内容的完整性,平台不提供额外的编辑、内容补充、找答案等附加服务。
- 2、"仅部分预览"的文档,不可在线预览部分如存在完整性等问题,可反馈申请退款(可完整预览的文档不适用该条件!)。
- 3、如文档侵犯您的权益,请联系客服反馈,我们会尽快为您处理(人工客服工作时间:9:00-18:30)。
Biologically-Inspired Design of Autonomous and Adaptive Grid ServicesChonho Lee and Junichi SuzukiDepartment of Computer ScienceUniversity of Massachusetts, Boston{chonho, jxs} @ Abstract—This paper describes and evaluates a bio-logically-inspired network architecture that allows grid ser-vices to autonomously adapt to dynamic environment changes in the network. Based on the observation that the immune system has elegantly achieved autonomous adapta-tion, the proposed mechanism, the iNet artificial immune system, is designed after the mechanisms behind how the immune system detects antigens (e.g., viruses) and specifi-cally reacts to them. iNet models a set of environment condi-tions (e.g., network traffic and resource availability) as an antigen and a behavior of grid services (e.g., migration and replication) as an antibody. iNet allows each grid service to autonomously sense its surrounding environment conditions (an antigen) to evaluate whether it adapts well to the sensed conditions, and if it does not, adaptively perform a behavior (an antibody) suitable for the sensed conditions. Simulation results show that iNet allows grid services to autonomously adapt their population and location to environmental changes for improving their performance (e.g., response time and throughput) and balancing workload.1. IntroductionGrid computing applications are expected to be autonomous and adaptive to dynamic environmental changes (e.g., workload surges) in order to improve user experience, expand application’s operational lon-gevity and reduce maintenance cost [1, 2, 3]. As inspi-ration for a new design paradigm for grid applications, we observe various biological systems have elegantly achieved autonomy and adaptability. We believe if grid applications are designed after certain biological concepts and mechanisms, they may be able to attain autonomy and adaptability.BEYOND1is a network architecture that applies key biological mechanisms to design autonomous and adaptive grid applications. In BEYOND, a grid appli-cation is modeled as a decentralized group of software agents. This is analogous to a bee colony (an applica-tion) consisting of multiple bees (agents). Each agent implements a functional service related to the applica-tion and follows biological behaviors such as migra-tion, replication, energy exchange and death.This paper focuses on an adaptation mechanism for agents (i.e., grid applications) to autonomously adapt to dynamic environmental changes. The proposed ad-aptation mechanism, iNet, is designed after the mecha-1 Biologically-Enhanced sYstem architecture beyond Ordinary Net-work Design nisms behind how the immune system detects antigens (e.g., viruses) and produces specific antibodies to kill them. iNet models a set of environment conditions (e.g., network traffic) as an antigen and a behavior of agents as an antibody. iNet allows each agent to autonomously sense its local environment conditions (an antigen) to evaluate whether it adapts well to the sensed conditions, and if it does not, adaptively per-form a behavior (an antibody) suitable for the condi-tions. For example, agents may invoke migration be-havior for moving towards network hosts that accept a large number of user requests for their services. This leads to the adaptation of agent locations, and agents can reduce response time for users. Simulation results show iNet allows agents to autonomously adapt to dy-namic environmental changes for improving their per-formance (e.g., response time and throughput) and balancing workload. This paper is organized as fol-lows: Section 2 overviews the design principles of BEYOND. Section 3 describes the design of iNet. Sec-tion 4 shows simulation results. Sections 5 and 6 con-clude with comparison with related work.2. BEYONDIn BEYOND, agents are designed based on the four principles described below.Decentralization: Agents are decentralized. There are no central entities to control and coordinate agents (i.e. no directory servers and no resource managers). Decentralization allows grid services to be scalable and simple by avoiding performance bottleneck and any central coordination in deploying them [4, 5].Autonomy: Agents are autonomous. Agents moni-tor their local network environments and autono-mously behave and interact without any interventions from/to other agents and human users.Adaptability: Agents are adaptive to dynamic envi-ronment conditions (e.g., user demands and resource availability). Each agent contains iNet, which allows it to adaptively behave against the current environment conditions (Figure 1).Symbiosis: Agents are grouped as species depend-ing on the services they provide. Different species (groups of agents) usually compete with each other for resources such as CPU cycles and memory space.However, in some circumstances, they share available resources to live together in a cooperative manner.Agents run (or live) on a middleware platform in a network host. Each platform provides a set of runtime services that agents use to perform their services and behaviors.Each agent consists of attributes, body and behav-iors. Attributes carry descriptive information regarding the agent (e.g., agent ID). The body implements a ser-vice the agent provides. For example, an agent may implement a genetic algorithm for an optimization problem, while another agent may implement a physi-cal model for scientific simulations. Behaviors imple-ment non-service related actions inherent to all agents. This paper focuses on the following six behaviors.Migration: Agents may move between platforms.Energy exchange and storage: Agents may receive and store energy in exchange for providing services to other agents or users. Agents may also expend energy for services that they receive from other agents, and for resources available on a platform (e.g. memory space).Communication: Agents may communicate with other agents for the purposes of, for example, request-ing a service or exchanging energy.Replications: Agents may make their copies in re-sponse to higher energy level, which indicates higher demand for the agents. A replicated agent is placed on the platform that its parent agent resides on, and it re-ceives the half amount of the parent’s energy level.Death: Agents die due to energy starvation. If en-ergy expenditure of an agent is not balanced with en-ergy gain, the agent cannot pay for the resources it needs; thus, it dies from lack of energy. When an agent dies, an underlying platform removes the agent and releases all resources allocated to the agent.Swapping: Different groups of agents (species) may cooperatively separate their locations (habitats) in the network when a group receives much larger number of service requests than another group. Agents in higher demand can ask other agents in lower demand to swap their locations. As a result, agents in higher demand can preferentially migrate towards users or certain re-sources; thereby increasing their performance (e.g., response time for users and throughput).3. The iNet Artificial Immune SystemThis section overviews how the natural immune system works (Section 3.1), and describes how the iNet artificial immune system is designed after the natural immune system (Section 3.2).3.1. Natural Immune SystemThe immune system is an adaptive defense mecha-nism to regulate the body against dynamic environ-ment changes (e.g. antigen invasions). Through a num-ber of interactions among various white blood cells (e.g. macrophages and lymphocytes) and molecules (e.g. antibodies), the immune system evokes two re-sponses to antigens: innate and adaptive immune re-sponse.In the innate immune response, the immune system performs self/non-self discrimination to detect antigens. This response is initiated by macrophages and T-cells, a type of lymphocytes. Macrophages move around the body to ingest antigens and present them to T-cells so that T-cells can recognize them. T-cells are produced in thymus and trained through the negative selection process. In this process, thymus removes T-cells that react with the body’s own (self) cells. The remaining T-cells are used as detectors to identify non-self cells (i.e. antigens). When T-cells detect non-self cells, they secrete chemical signals to activate the second immune response: adaptive immune response.In the adaptive immune response, the immune sys-tem produces antibodies that specifically react and kill an antigen identified by T-cells. Antibodies form a net-work structure and communicate with each other [6]. This network is formed with stimulation and suppres-sion relationships among antibodies. Thus, the adap-tive immune response is offered by multiple types of antibodies, although a single type of antibody (the best matched with an antigen) may play the dominant role. The immune network also helps to keep the quantita-tive balance of antibodies. Through the stimulation and suppression interactions, the population of specific antibodies rapidly increases following the recognition of an antigen and, after eliminating the antigen, de-creases again. Performed based on this self-regulation mechanism, the adaptive immune response is an emer-gent product from many interactions among antibodies.3.2. Design and Implementation of iNetThe iNet artificial immune system consists of the environment evaluation (EE) facility and behavior selection (BS) facility (Figure 1) corresponding to the innate and adaptive immune response, respectively. EE allows each agent to continuously sense a set of cur-rent environment conditions as an antigen and examinethat the agent adapts to the current environment condi-tions well, and a non-self antigen indicates it does not. When EE detects a non-self antigen, EE activates BS (Figure 1). BS allows each agent to choose a behavior as an antibody that specifically matches with the de-tected non-self antigen.3.2.1. Environment Evaluation Facility (EE)EE performs two steps: initialization and self/non-self classification (Figure 2). The initialization stepproduces detectors that identify self and non-self anti-gens (i.e. environment conditions). In iNet, an antigen (i.e. a set of environment conditions) is implemented as a feature vector. Each feature vector (X) consists of a set of features (F) and a class value (C). F contains a series of environment conditions. If an agent senses agent population on a local platform, resource utiliza-tion on a local platform and workload (the number of user requests) on a local platform, a feature vector may be represented such as X curent=((Low: Agent population, Low: Resource utilization, Heavy: Workload), C). C indicates whether a given antigen (i.e. a set of environ-ment conditions) is self (0) or non-self (1).To evaluate whether an antigen (i.e. feature vector) is self or non-self, the initialization step produces de-tectors that identify it (Figure 2). This step is designed after the negative selection process in the immune sys-tem. In the initialization step, EE first generates feature vectors randomly, and separates them into self detec-tors, which closely match with self antigens (feature vectors), and non-self detectors (T-cells in the immune system), which do not closely match with self antigens (feature vectors). This separation is performed via vec-tor matching between randomly generated feature vec-tors and self antigens that human users supply (Figure 3). Currently, EE uses the Euclidean vector matching algorithm. After vector matching, both self and non-self detectors are stored in a feature table (Figure 3)2.2 The immune system removes non-self detectors through negative selection process. However, in iNet, both self and non-self detectors are kept in a feature table to perform self/non-self classification. fication of environment conditions (Figure 2). It uses the detectors in a feature table to classify the current environment conditions into self or non-self. The self/non-self classification step is performed with a decision tree built from detectors in a feature table. Figure 4 shows an example decision tree. EE starts to examine a set of given current environment conditions, X current, at the root of the decision tree. Each node in the tree specifies which feature is considered. Based on the value of the specified feature in X current, EE follows down along the branch indicating the value. This proc-ess is repeated until EE reaches at the leaf of tree which notices the class value of X current. Once EE de-tects a non-self antigen, it activates BS immediately.The reasons for using decision tree as a classifier are ease of implementation and algorithmic efficiency. Since a decision tree is easy to understand and imple-ment, iNet can maintain a lower barrier for developers to design adaptive grid applications. Also, a decision tree performs classification much faster than other al-gorithms such as clustering, support vector machine and Markov model algorithms [7, 8]. The efficiency of classification is one of the most important require-ments in iNet because each agent periodically senses and classifies its surrounding environment conditions.3.2.2. Behavior selection facility (BS)Once EE classifies the current environment condi-tions as a non-self antigen, it activates BS. BS selects an antibody (i.e. agent’s behavior) suitable for the de-tected non-self antigen (i.e. environment conditions).Each antibody is structured as shown in Figure 5. It consists of Paratope , precondition under which it is selected (one of environment conditions), Behavior ID , one of agent behaviors, and Idiotope , relationships to other antibodies (one or more links). Antibodies are linked with each other using stimulation and suppres-sion relationships (see Section 3.1). Each antibody has its own concentration value corresponding to the num-ber of the antibody. The value is used to prioritize anti-bodies (behaviors). BS identifies candidate antibodies (behaviors) suitable for a given non-self antigen (envi-ronment conditions), prioritizes them based on their concentration values, and selects the most suitable one from the candidates. When prioritizing antibodies (be-haviors), stimulation relationships between them con-tribute to increase their concentration values, and sup-pression relationships contribute to decrease it. Each relationship has its own strength (affinity), which indi-cates the degree of stimulation or suppression.Figure 6 shows a generalized network of antibodies.N antibodies. m ji and m ik denote affinity values be-tween antibody j and i , and between antibody i and k . m i is an affinity value between an antigen and antibody i . The concentration of antibody i , denoted by a i , is calculated with the following equations.(2) ..... ))(5.0exp(11)((1) ... )(1)(1)(11)(t i A t i a t i a k i m Mk t k a ik m M t j a N j ji m N dtt i dA −+=−+∑=⋅−⋅∑==⎟⎟⎠⎞⎜⎜⎝⎛In the equation (1), the first and second terms in a big bracket denote the stimulation and suppression from other antibodies. The affinity values between antibodies (i.e. m ji and m ik ) are positive between 0 and 1. m i is 1 when antibody i is stimulated directly by an antigen, otherwise 0. k denotes the dissipation factor representing the natural death of an antibody. This value is 0.1. The initial concentration value for every antibody, a i (0), is 0.01. The equation (2) is a sigmoid function used to squash the A i (t) value between 0 and 1. Every antibody’s concentration is calculated 200 times repeatedly. This repeat count is obtained from a previous simulation experience [9, 10]. If no antibody exceeds a predefined threshold (0.7) during the 200value is the highest is selected (i.e. winner-tales-all selection). If one or more antibodies’ concentration values exceed the threshold, an antibody is selected based on the probability proportional to the current concentrations (i.e. roulette-wheel selection).Figure 7 shows an example network of antibodies. It contains four antibodies, which represent migration, replication, swapping and death behaviors. Antibody 1 represents the migration behavior invoked when dis-tance to users is far. Antibody 1 suppresses Antibody 3 when it is stimulated Now, suppose that a (non-self) antigen indicates (1) user location is far, (2) network traffic is heavy on the local platform and (3) resource availability is high at local platform. This antigen stimulates Antibodies 1, 2 and 4 simultaneously. Their population increases, and it is likely that Antibody 2’s concentration value becomes highest because Anti-body 2 suppresses Antibody 4, which in turn sup-presses Antibody 1. As a result, Antibody 2 (i.e. repli-cation behavior) would be selected.4. Simulation ResultsThis section shows simulation results to examine the autonomous adaptability of agents developed with iNet. The simulations are carried out on the BEYOND simulator 3. Figure 8 shows a simulated network. A server farm consists of network hosts connected in a 15 x 15 grid topology, and service requests travel from users to agents via user access point. This simulation study assumes that a single (emulated) user runs on the access point and sends service requests to agents.3The BEYOND simulator contains 13,490 lines of Java code. It is available for researchers who investigate autonomous and adaptive grid applications ().quest is passed to the local platform where the user resides on, and the platform performs a discovery process to search a target agent that can process the issued service request. The platform (discovery origi-nator) forwards a discovery message to its neighboring platforms, asking whether they host a target agent. If a neighboring platform hosts a target agent, it returns a discovery response to the discovery originator. Other-wise, it forwards the discovery message again to its neighboring platforms. Figure 9 shows pseudo code for this agent discovery through platform connectivity4. While (not simulation last cycle)If ( Discovery messages arrived)For each of discovery msgs (under the max # of messages to be processed in each simulation cycle) DoIf ( Discovery message matches one of the local agents)Returns a discovery response to discovery originatorElseForward the discovery message to neighboring platforms End IfEnd ForEnd IfEnd WhileFigure 9. Pseudo Code for Agent Discovery Processin each Simulation Cycle4.1. Local-Area GridIn the first simulation study, each agent implements web server functionality in its body and possesses an iNet immune network that implements three behaviors (migration, replication and death), five environment conditions (energy level, workload on the local/ neighboring platforms, and resource availability on the local/neighboring platforms), 10 antibodies and 7 stimulation/suppression relationships. An assumed application in this simulation study is web servers hosted on a server cluster. At the beginning of simula-tion, a single agent is deployed on a platform at the right bottom corner of server farm (Figure 8).Figure 10 (1) (All figures are shown in the last page.) shows how service request rate changes over time. It starts with 1000 requests/min, spikes to 60,000 requests/min at 2:00, and drops to 1,000 requests/min at 5:00. The peak-to-trough ratio is 60:1. This ratio is designed based on a workload trace of the 1998 World Cup official web site [16].4 Note that there is no centralized directory to keep track of agents. Figure 10 (2) shows how agents autonomouslyworkload spikes, agents gains more energy from users and perform replication behavior to increase their population. On the other hand, when the workload drops, some agents die due to energy starvation be-cause they cannot balance their energy gain from users and energy expenditure to utilize the resources. As a result, agents shrink their population by eliminating idle ones.Figure 10 (3) shows how agents autonomously adapt response time for a user. At the beginning of simulation, response time becomes very high because only one agent processes 1,000 requests a minute and distance between the agent and users is long. However, after the agents accumulate enough energy from users and start migrating towards users and replicating them-selves, they rapidly decrease response time (less than a second). When workload spikes at 2:00, the response time spikes up to 14 seconds (Figure 10 (4)), but agents decrease it to 1 second in 20 minutes by per-forming replication behavior according to the demand change.Figure 10 (5) depicts the average distance between agents and users. Initially, a single agent is placed at the right bottom corner, and users are at the left most (Figure 8). Therefore, the initial distance between agents and users is 22. Agents autonomously decrease the distance by performing migration behavior.Figure 10 (6) shows the throughput achieved by agents. It is measured as the number of responses that users receives a minute from agents. Figure 9 demon-strates that agents autonomously meet given workload by dynamically adjusting their population and loca-tions through replication and migration behaviors.4.2. Wide-Area GridThe second simulation study is carried out to evalu-ate how agents autonomously balance workload placed on them and how symbiosis among agents (swapping behavior) impact agent performance. In this study, each agent implements multimedia streaming function in its body and possesses an iNet immune network that implements three behaviors (migration, replication, death and swapping), five environment conditions (en-ergy level, workload on the local/neighboring plat-forms, and resource availability on the lo-cal/neighboring platforms), 13 antibodies and 10 stimulation/suppression relationships.An assumed application in this simulation study is multimedia content distribution in a grid (a collection of servers) that covers a geographically wide area. For example, a large sport event such as Olympic game may deploy servers at multiple event sites such as ath-letics stadiums, swimming pools and gymnasiums. The servers are connected with each other to form a wide-area grid. Each event site has a wireless base station connected to this grid, and spectators in the event site can access realtime zoom-in video or playback video. This simulation study assumes that 15x15 (225) serv-ers are deployed, each agent contain a multimedia steam (or a fragment of the stream), and user access point is a wireless base station through which users access agents for multimedia contents.In this simulation, there are two types of agents that provide different types of multimedia contents: A and B. At the beginning of simulation, three agents for each service A and B are randomly deployed in the network. Figure 11 shows dynamic changes of service request rate for service A and B. Service A is con-stantly requested at the rate of 25K requests/min from 0:00 to 2:00. This simulates that spectators in an ath-letic stadium constantly request playback movie of an athletic match. At 2:00, service request rate spikes from 0 to 35K requests/min. This simulates that the spectators in an athletic stadium rapidly access the movie of a swimming final match that generated the world record.Figure 12 shows how a swapping behavior effec-tively works in terms of response time for users. The black line indicates response time by agents with swapping behavior, and the gray line indicates re-sponse time by agents without swapping behavior. The first graph in Figure 11 shows that response time isslightly increasing (about 0.4sec) because agents for service A yield their resources to agents for service B. However, the second graph in Figure 11 shows that response time drops about 4 sec since agents for ser-vice B gain more resources by swapping behavior. So, the total average response time also reduces. As pre-sented in section 2, a swapping behavior is performed between two different types of agents. Agents compare their workload with others, and if the workload is heavier then they try to swap their location and gain resources closer to users.Figure 13 shows that how agents dynamically dis-tribute their workload over the simulated grid network.X i indicates the current workload of platform i per a resource unit (i.e. the # of messages processed by agents running on platform i divided by resource utili-zation on platform i). µrepresents the expected aver-age of X (i.e. the total # of messages processed by all agents divided by the total amount of resource utiliza-tion on all platforms N where those agents are run-ning.) Hence, LBI represents a variance of workload on each platform. Figure 13 shows that LBI is decreas-ing from 2:00 to 3:00. This indicates that, when agents receive service B requests at 2:00, agents start replicat-ing, and the workload is being distributed over the network.Figure 14 describes how each group of agents (i.e. agents for service A and B) forms a cluster when they migrating toward users. Figure 14 (A) shows the clus-ter size of agents for service A. At 0:00, three agents are deployed and form a cluster with size 10. When they receive requests from users, they migrate toward users and reduce cluster size to about 2.5. Similarly, figure 14 (B) shows the cluster size of agents for ser-vice B. When they receive requests at 2:00, they start migrating toward users from up, right and bottom (due to initial location). At 2:15, they can no longer migrate (with cluster size 8) because of a boundary of agents for service A which are already consuming resources closer to users. However, agents for service B invoke swapping behavior and get close to each other (with cluster size 6) at 3:00. Swapping behavior helps to reduce cluster size of the same type of agents.5. Related workArtificial immune systems have been proposed and used in various application domains such as anomaly detection [11] and pattern recognition [12]. [11] mainly focuses on the generation of detectors forselection process of the artificial immune system. [12] focuses on the accuracy for the matchmaking of an antigen and antibody. On the other hand, this paper proposes an artificial immune system to improve autonomous adaptability in grid network services. To the best of our knowledge, this work is the first attempt to apply an artificial immune system into the auto-nomic adaptation domain.There are lots of research efforts to achieve decen-tralized network applications based on the concept of natural immune system. For example, [17] uses the concept of memory B-cells to reduce search response time. Once the target for query is found, B-cell (user node) remembers the query and the peers (nodes) who contain the target. They move close each other and form clusters so that reducing response time for the query encountered before. [18] follows the concept of stimulation/suppression interaction among antibodies to find the recommending items that have been never seen before by users. Stimulation affects to increase the concentration of similar items to a user profile as recommending items; suppression affects to keep items that have seen before by users far away from the rec-ommending items. Similar to [17][18], iNet agent in-cludes the concept of B-cells such as the network of antibodies and the memory of antibody’s concentrationin the BS facility. However, the iNet agent also in-cludes the function of T-cells such as self/non-self discrimination in the EE facility. This additional func-tion results in reducing the overhead of behavior selec-tion.Similar work has been proposed in Organic Grid [13] project. [13] attempts to the decentralized task scheduling for large-scale computation on grid envi-ronment over the Internet. Similar to iNet, mobile agents autonomously executes their services (e.g. com-puting subtasks) on the platform embedded in each host and perform their replication behavior to achieve their objectives (e.g. compute as fast as possible). Yet, iNet focuses on the grid service adaptation. Agents consider more behaviors for adaptation, and through those various adaptation decisions, the high adaptabil-ity of grid network services (i.e. agents) is achieved.There are several research efforts that allow net-work systems to adapt to application and end-user re-quirements with a technique of runtime component replacement. For example, [14, 15] can dynamically replace running components (e.g. byte code) with oth-ers according to the monitored environment conditions. The difference is that [14, 15] assumes a centralized network architecture where a centralized server col-lects environment conditions from each component to make replacement decisions. In contrast, iNet assumes a decentralized network architecture where each agent monitors its surrounding environment and makes adap-tation decisions.6. Concluding RemarksThis paper describes and evaluates a biologically-inspired mechanism that allows grid services to autonomously adapt to dynamic changes in the net-work. The proposed adaptation mechanism, called the iNet artificial immune system, allows each grid service to autonomously sense its surrounding environment conditions to evaluate whether it adapts well to the conditions, and if it does not, adaptively perform a behavior suitable for the conditions. Simulation results show that iNet allows agents to autonomously adapt to dynamic environmental changes for improving their performance (e.g., response time and throughput) and balancing workload.7. References[1] P. Dini, W. Gentzsch, M. Potts, A. Clemm, M. Yousifand A. Polze, “Internet, Grid, Self-adaptability and Be-yond: Are We Ready?,” In Proc. of the IEEE Int’l Workshop on Self-Adaptable and Autonomic ComputingSystems, Aug. 2004.[2] Large Scale Networking Coordinating Group of theInteragency Working Group for Information Technol-ogy Research and Development (IWG/IT R&D), Reportof Workshop on New Visions for Large-scale Networks: Research and Applications, Mar 2001.[3] R. Sterritt and D. Bustard, “Towards an AutonomicComputing Environment,” In Proc. of 14th IEEE Inter-national Workshop on Database and Expert Systems Applications, Sep 2003.[4] N. Minar, K. H. Kramer and P. Maes, “CooperatingMobile Agents for Dynamic Network Routing,” In A.Hayzelden and J. Bigham (eds.) Software Agents for Fu-ture Communications Systems, Springer, 1999[5] G. Cabri, L. Leonardi and F. Zambonelli, “Mobile-Agent Coordination Models for Internet Applications,”In IEEE Computer, Feb 2000.[6] N.K.Jerne, “Idiotypic Networks and Other PreconceivedIdeas,” In Immunological Review, vol. 79, 1984.[7] P. Berkhin, “Survey of Clustering Data Mining Tech-niques,” Accrue Software, Inc., San Jose, CA, 2002.[8] T. Mitchell, Machine Learning, McGraw-Hill, 1997.[9] J. Suzuki and Y. Yamamoto, “iNet: An ExtensibleFramework for Simulating Immune Network,” In Proc.of IEEE International Conference on Systems, Man, and Cybernetics, October 2000.[10] J. Suzuki and T. Suda, “Adaptive Behavior Selection ofAutonomous Objects in the Bio-Networking Architec-ture,” In Proc. of 1st Annual Symposium on Autonomous Intelligent Networks and Systems, May 2002.[11] F. A. González, D. Dasgupta, “Anomaly Detection Us-ing Real-Valued Negative Selection,” Genetic Program-ming and Evolvable Machines, 4(4), 2003.[12] L. N. de Castro, J. I. Timmis, “Artificial Immune Sys-tems: A Novel Paradigm to Pattern Recognition,” In Ar-tificial Neural Networks in Pattern Recognition, J. M.Corchado, L. Alonso, and C. Fyfe (eds.), SOCO-2002, University of Paisley, UK, pp. 67-84.[13] A.J. Chakravarti, G. Baumgartner, M. Lauria, “TheOrganic Grid: Self-organizing Computational Biology on Desktop Grid,” In A Zomaya, Parallel Computing for Bioinformatics, John Wiley & Sons, 2005.[14] S. Cheng, D. Garlan, B. Schmerl, P. Steenkiste, and N.Hu, “Software Architecture-based Adaptation for Grid Computing,” In the 11th IEEE Conference on High Per-formance Distributed Computing, July 2002.[15] K Shirose, S Matsuoka, H Nakada, and H Ogawa,“Autonomous Configuration of Grid Monitoring Sys-tems,” In the 2004 Symposium on Application and the Inte rnet (SAINT2004), Japan, January 2004.[16] Martin Arlitt and Tai Jin, “A Workload CharacterizationStudy of the 1998 World Cup Web Site,” IEEE Net-work, Vol. 14, No. 3, pp. 30-37, May/June 2000. [17] N. Ganguly, G. Canright and A. Deutsch, “Design of anEfficient Search Algorithm for P2P Networks Using Concepts from Natural Immune Systems,” In proc of Parallel Problem Solving from Nature 2004, 491-500. [18] S. Cayzer1 and U. Aickelin, “A Recommender Systembased on the Immune Network,” In proc of Congress on Evolutionary Computation 2002, Honolulu, USA, 2002.。