PeakTime参考文件-商业模拟课件-2007PT2
AnaChip AP2007 数据手册
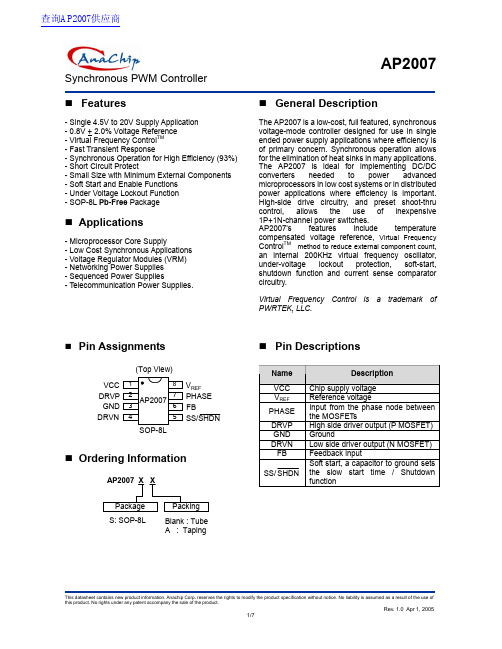
AP2007Synchronous PWM ControllerFeatures- Single 4.5V to 20V Supply Application - 0.8V + 2.0% Voltage Reference - Virtual Frequency Control TM - Fast Transient Response- Synchronous Operation for High Efficiency (93%) - Short Circuit Protect- Small Size with Minimum External Components - Soft Start and Enable Functions - Under Voltage Lockout Function - SOP-8L Pb-Free PackageApplications- Microprocessor Core Supply- Low Cost Synchronous Applications - Voltage Regulator Modules (VRM) - Networking Power Supplies - Sequenced Power Supplies- Telecommunication Power Supplies.General DescriptionThe AP2007 is a low-cost, full featured, synchronous voltage-mode controller designed for use in single ended power supply applications where efficiency is of primary concern. Synchronous operation allows for the elimination of heat sinks in many applications. The AP2007 is ideal for implementing DC/DC converters needed to power advanced microprocessors in low cost systems or in distributed power applications where efficiency is important. High-side drive circuitry, and preset shoot-thru control, allows the use of inexpensive 1P+1N-channel power switches.AP2007’s features include temperature compensated voltage reference, Virtual Frequency Control TM method to reduce external component count, an internal 200KHz virtual frequency oscillator, under-voltage lockout protection, soft-start, shutdown function and current sense comparator circuitry.Virtual Frequency Control is a trademark of PWRTEK, LLC.Pin AssignmentsSOP-8L1(Top View)VCC V REFPHASE DRVP DRVNFBGND AP20072345678SS/SHDNOrdering InformationAP2007 X X Package Packing S: SOP-8LBlank : Tube A : TapingPin DescriptionsNameDescriptionVCC Chip supply voltage V REF Reference voltagePHASEInput from the phase node betweenthe MOSFET sDRVP High side driver output (P MOSFET)GND Ground DRVN Low side driver output (N MOSFET) FB Feedback inputSS/SHDN Soft start, a capacitor to ground setsthe slow start time / Shutdownfunction查询AP2007供应商Synchronous PWM ControllerBlock DiagramCROSS CURRENT CONTROL DRVNVIRTUAL FREQ OSCILLATORDRVPR Q SQSQB R+-+-+-+-VOLTAGE REFERENCE +-VCC0.8VUNDER VOLTAGEERROR COMPVCC 12ua2ua0.2V0.9VSS/SHDNFBGNDOCSETPHASEVCCDRVPDRVNAP2007 FUNCTIONAL BLOCK DIAGRAMVirtual Frequency Control - PatentNumber 6,456,050.V REF-+0.4V -+0.4VAbsolute Maximum RatingsSymbol ParameterRange. Unit V IN VCC to GND -1 to 22 V V PHASE PHASE to GND -1 to 22 V V DRVP DRVP to GND -1 to 22 V V DRVN DRVN to GND-1 to 22 V θJC Thermal Resistance Junction to Case 90 oC/W θJA Thermal Resistance Junction to Ambient 250 oC/W T OP Operating Temperature Range -40 to +85 o C T ST Storage Temperature Range-65 to +150o C T LEADLead Temperature (Soldering) 10 Sec.300o CSynchronous PWM ControllerElectrical CharacteristicsUnless specified: V CC =12V; GND = 0V;V O = 5V; T J = 25oCSymbol Parameter Conditions Min. Typ. Max. Unit Power SupplyV CC Supply Voltage(Recommended)4.5 - 20 VI CC Supply Current DRVP & DRVN are floating - 9.5 - mA ∆V LINE Line Regulation V O = 2.5V - 0.5 % Error Comparator A OL Gain (A OL ) - 70 - dB I B Input Bias - 0.2 1 uA Oscillator F OSC Oscillator Frequency - 200 - KHz DC MAX Oscillator Max Duty Cycle 80 85 - % Mofset DriversI DRVP DRVP Source/Sink V CC – V DRVP =3VV DRVP – V GND = 2V 0.5 1 - AI DRVN DRVN Source/Sink V CC – V DRVN = 3VV DRVL – V GND = 2V0.5 1 - AV DRVL DRVP/N Low Level Voltage - - 1.2 V V DRVH DRVP/N High Level Voltage V CC -1.2- - V ProtectionT DEAD Dead Time DRVP & DRVN are floating - 150 - nS Vocset Over Current Setting Voltage 0.4 VV DRVP/N DRVP/DRVN System ErrorVoltage (Note3) V SS =Low, V CC <3.8, over current happenV CC -1.2- - VReferenceReference Voltage 0.7840.8 0.816V V REF Accuracy 0o C to 70oC-2 - + 2 %Soft StartI SSC Charge Current V SS = 1.5V 8.0 10 12 uA I SSD Discharge Current V SS = 1.5V 1.3 2 2.7 uA Under voltage lockout (UVLO)V UT Upper Threshold Voltage (V CC )- 4.0 - V V LWT Lower Threshold Voltage (V CC )- 3.8 - V V HT Hysteresis (V CC ) - 200 - mVNote 1. Specification refers to Typical Application Circuit.Note 2. This device is ESD sensitive. Use of standard ESD handling precautions is required. Note 3. Abnormal condition; Ex: over-current, under-voltage lockout, soft-start disappear.Synchronous PWM ControllerTypical Application Circuit87651234D1Option VCC SS/SHDN FB DRVP GNDPHASE DRVN Q1Q2L110uHC8470u/16V C9Vout=3.2V*+-+-C1R21KR33K ** Vout = 0.8 x (1+R3/R2)AP2007C4330nC3330nR112ΩV REF 10n470u/16V470u/16VAF9435C5AF9410C20.1uC647n C70.1u 1ΩOption 1ΩOptionR2 1K ~ 10K≅(4835)(4412)Virtual Frequency ControlVirtual Frequency Control combines the advantages of constant frequency and constant off-time control in a single mode of operation. This allows fix frequency, precision switching voltage regulator control with fast transient response and the smallest solution size. Switch duty cycle can be adjusted from 0% to 100% on a pulse by pulse basis when responding to transient conditions. Both 0% and 100% duty cycle operation can be maintained for extended periods of time in response to load or line transients. Figure 1 depicts a simplified operation of the Virtual Frequency Controltechnique: The VFC oscillator generates a pulse of a known duration (VFC_Pulse). The regulator loop responds by returning a complementary feedback pulse (FB_Pulse). The FB_Pulse duration is a result of external conditions such as inductor size, the voltage across the inductor and the duration of the VFC_Pulse. A VFC control loop is then formed whereby the duration of the VFC_Pulse is modified as a result of the FB_Pulse duration. The VFC loop arrives at a state of equilibrium, where the operating frequency remains inherently constant.GATE CONTROL LOGICVIRTUAL FREQ OSCILLATOR+-FB PulseVFC PulseVrefERROR COMPV INLout CoutVout Rfb1Rfb2Figure 1: Virtual Frequency Control Loop- Synchronous single supply application.Synchronous PWM with VFC Controller (Preliminary) Virtual Frequency Control (Continued)Virtual frequency control is a technique that provides stable, constant frequency of operation for pulse controlled architectures such as constant off-time/on-time. This is all done internal to the IC with minimal number of components and without the need for connections to external terminals such as input and/or output. No external compensation is required, thus providing a low cost, high performance fix frequency solution for switching voltage regulators.Virtual Frequency Control is a trademark of PWRTEK, LLC.Function DescriptionSynchronous Buck ConverterPrimary V CORE power is provided by a synchronous, voltage-mode pulse width modulated (PWM) controller. This section has all the features required to build a high efficiency synchronous buck converter, including soft-start, shutdown, and cycle-by-cycle current limit.Referring to the functional block diagram FIG 1, the output voltage of the synchronous converter is set and controlled by the output of the error comparator. The external resistive divider reference voltage, is derived from an internal trimmed-bandgap voltage reference. The inverting input of the error comparator receives its voltage from the FB pin.The internal oscillator uses an on-chip capacitor and trimmed precision current sources to set the virtual oscillation frequency to 200KHz. The virtual frequency oscillator sets the PWM latch. This pulls DRVN low, turning off the low-side N_MOSFET and DRVP is pulled low, turning on the high-side P-MOSFET (once the cross-current control allows it). The triangular voltage ramp at the FB pin is then compared against the reference voltage at the inverting input of the error comparator. When the FB voltage increases above the reference voltage, the comparator output goes high. This pulls DRVP high, turning off the high-side P-MOSFET, and DRVN is pulled high, turning on the low-side N-MOSFET (once the cross-current control allows it). The Virtual Frequency Oscillator then generates a programmed off time to allow the FB voltage to return to the valley voltage of the triangular ramp. At the end of the off time the PWM latch is set and the cycle repeats again.Under Voltage LockoutThe under voltage lockout circuit of the AP2007 assures that the high-side P-MOSFET driver outputs remain in the off state whenever the supply voltage drops below set parameters. Lockout occurs if V CC falls below 3.8V. Normal operation resumes once V CC rises above 4.0V. R DS(ON) Current LimitingThe current limit threshold (0.4V) is set by connecting an internal resistor from the V CC supply to OCSET. Vocset is compared to the voltage at the PHASE node. This comparison is made only when the high-side drive is high to avoid false current limit triggering due to uncontributing measurements from the MOSFET s off-voltage. When the voltage at PHASE is less than the voltage at OCSET, an over-current condition occurs and the soft start cycle is initiated. The synchronous switchturns on and SS/SHDN starts to sink 2uA. When SS/ SHDN reaches 0.2V, it then starts to source 10uA and a new cycle begins. When the soft start voltage is below 0.9V the cycle is controlled with pulse by pulse current limiting.Soft StartInitially, SS/SHDN pin sources 10uA of current to charge an external capacitor. The inverting input of the error comparator is clamped to a voltage proportional to the voltage on SS/SHDN. This limits the on-time of the high-side P-MOSFET, thus leading to a controlled ramp-up of the output voltages.Synchronous PWM with VFC Controller (Preliminary)Function Description (Continued)Hiccup ModeDuring power up, the SS/SHDN pin is internally pulled low until V CC reaches the under-voltage lockout level of 4V. Once V CC has reached 4V, the SS/SHDN pin is released and begins to source 10uA of current to the external soft-start capacitor. As the soft-start voltage rises, the inverting input of the error comparator is clamped to this voltage. When the error signal reaches the level of the internal 0.8V reference, the output voltage is to have reached its programmed voltage. If an over-current condition has not occurred the soft-start voltage will continue to rise and level off at about 2.5V.An over-current condition occurs when the high-side drive is turned on, but the PHASE node does not reach the voltage level set at the OCSET pin. Once an over-current occurs, the high-side drive is turned off and the low-side drive turns on and the SS/SHDN pin begins to sink 2uA. The soft-start voltage will begin to decrease as the 2uA of current discharge the external capacitor. When the soft-start voltage reaches 0.2V, the SS/SHDN pin will begin to source 10uA and begin to charge the external capacitor causing the soft-start voltage to rise again. If the over-current condition is no longer present, normal operation will continue. If the over-current condition is still present, the SS/SHDN pin will again begin to sink 2uA. This cycle will continue indefinitely until the over-current condition is removed.In order to prevent substrate glitching, a small-signal diode should be placed in close proximity to the chip with cathode connected to PHASE and anode connected to GND.Marking Information(Top View)SOP-8L184AP2007YY WW XLogo"02" =2002~5Synchronous PWM ControllerPackage InformationPackage Type: SOP-8LVIEW "A"LHECVIEW "A"AA 2A 1B e D7(4X)0.015x457(4X)yDimensions In Millimeters Dimensions In Inches SymbolMin. Nom. Max. Min. Nom. Max.A 1.40 1.60 1.75 0.055 0.063 0.069 A1 0.10 - 0.25 0.040 - 0.100 A2 1.30 1.45 1.50 0.051 0.057 0.059B 0.33 0.41 0.51 0.013 0.016 0.020C 0.19 0.20 0.25 0.0075 0.008 0.010D 4.80 5.05 5.30 0.189 0.199 0.209E 3.70 3.90 4.10 0.146 0.154 0.161 e - 1.27 - - 0.050 - H 5.79 5.99 6.20 0.228 0.236 0.244 L 0.38 0.71 1.27 0.015 0.028 0.050 y - - 0.10 - - 0.004θ0O - 8O 0O- 8O。
TAKTTime节拍管理培训PPT学习课件
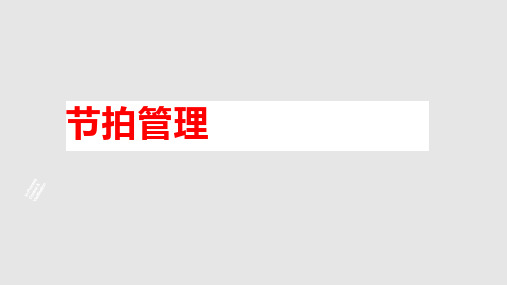
生产线速检测
1)检测线速与目标线速的误差为±3秒。 2)维修人员(或指定人员)每天每个班次(涂装为每天)需对生产线线速进行一次定期检测,每次以调整线连续三个车位 通过同一固定检测点的时间为一个检测节点,将检测结果记录在《生产线线速检测表》的“检测值”栏,并在“检测者” 栏签名。 3)如果检测结果符合目标线速范围,则在“调整后线速”划“/”。 4)如果检测结果与目标线速的误差超过规定值,检测人员应立即调整到目标线速,并在“调整后线速”栏记录。 5)生产值班长应每周检查生产线线速检测情况,并签字。
8)《生产线线速检测表》由维修/操作工段保存,《制造部线速检测表》由制造部保存,保存期1 年以上。
GMS/STD.32《生产线线速管理办法》
线速变更流程
1、若没有恒定的生产节拍,标准化则 无法实现.
2、 TAKT Time 在某一时期内是固定不变的! TAKT Time随意改变—安全、质量、效率就会出 问题!
工厂线速ATT变更
由工厂厂长系统规划ATT,每月评估/回顾ATT。按照变更程序,制定工厂、 车间/部门(生产、设备、物流、质量等)、工段/班组ATT变更计划,一般有 20天准备,包括岗位技能培训(改变SOS/JIS,线速维持不变),经过工位准 备状态验证,所有准备工作完成后才变更生产线线速ATT!
申 请 人: 审 核:
编 号:
申请日期:
拟变更 日 期:
评审意见:
评审会签: □生产计划: □技术中心: □质量部: □东部总装: □冲压车间: □西部车身:
批准:
评审组织人:
日 期:
□销售公司: □采购物流部: □I T 部:
TL072

PACKAGING INFORMATIONOrderableDevice Status (1)Package TypePackage DrawingPins Package Qty Eco Plan (2)Lead/Ball FinishMSL Peak Temp (3)8102304HA OBSOLETE 10TBD Call TI Call TI81023052A ACTIVE LCCC FK 201TBD POST-PLATE Level-NC-NC-NC8102305HA ACTIVE CFP U 101TBD A42SNPB Level-NC-NC-NC 8102305PA ACTIVE CDIP JG 81TBD A42SNPB Level-NC-NC-NC 81023062A ACTIVE LCCC FK 201TBD POST-PLATE Level-NC-NC-NC8102306CA ACTIVE CDIP J 141TBD A42SNPB Level-NC-NC-NC 8102306DA ACTIVE CFP W 141TBD A42SNPB Level-NC-NC-NC JM38510/11905BPA ACTIVE CDIP JG 81TBD A42SNPB Level-NC-NC-NC JM38510/11906BCAOBSOLETE CDIP J 14TBD Call TI Call TITL071ACD ACTIVE SOIC D 875Green (RoHS &no Sb/Br)CU NIPDAU Level-1-260C-UNLIM TL071ACDE4ACTIVE SOIC D 875Green (RoHS &no Sb/Br)CU NIPDAU Level-1-260C-UNLIM TL071ACDR ACTIVE SOIC D 82500Green (RoHS &no Sb/Br)CU NIPDAU Level-1-260C-UNLIM TL071ACDRE4ACTIVE SOIC D 82500Green (RoHS &no Sb/Br)CU NIPDAU Level-1-260C-UNLIM TL071ACP ACTIVE PDIP P 850Pb-Free (RoHS)CU NIPDAU Level-NC-NC-NC TL071ACPE4ACTIVE PDIP P 850Pb-Free (RoHS)CU NIPDAU Level-NC-NC-NC TL071BCD ACTIVE SOIC D 875Green (RoHS &no Sb/Br)CU NIPDAU Level-1-260C-UNLIM TL071BCDE4ACTIVE SOIC D 875Green (RoHS &no Sb/Br)CU NIPDAU Level-1-260C-UNLIM TL071BCDR ACTIVE SOIC D 82500Green (RoHS &no Sb/Br)CU NIPDAU Level-1-260C-UNLIM TL071BCDRE4ACTIVE SOIC D 82500Green (RoHS &no Sb/Br)CU NIPDAU Level-1-260C-UNLIM TL071BCP ACTIVE PDIP P 850Pb-Free (RoHS)CU NIPDAU Level-NC-NC-NC TL071BCPE4ACTIVE PDIP P 850Pb-Free (RoHS)CU NIPDAU Level-NC-NC-NC TL071CD ACTIVE SOIC D 875Green (RoHS &no Sb/Br)CU NIPDAU Level-1-260C-UNLIM TL071CDE4ACTIVE SOIC D 875Green (RoHS &no Sb/Br)CU NIPDAU Level-1-260C-UNLIM TL071CDR ACTIVE SOIC D 82500Green (RoHS &no Sb/Br)CU NIPDAU Level-1-260C-UNLIM TL071CDRE4ACTIVE SOIC D 82500Green (RoHS &no Sb/Br)CU NIPDAU Level-1-260C-UNLIM TL071CP ACTIVE PDIP P 850Pb-Free (RoHS)CU NIPDAU Level-NC-NC-NC TL071CPE4ACTIVE PDIP P 850Pb-Free (RoHS)CU NIPDAU Level-NC-NC-NC TL071CPSR ACTIVE SO PS 82000Green (RoHS &no Sb/Br)CU NIPDAU Level-1-260C-UNLIM TL071CPSRE4ACTIVESOPS82000Green (RoHS &CU NIPDAULevel-1-260C-UNLIM17-Oct-2005OrderableDeviceStatus (1)Package Type Package Drawing Pins Package QtyEco Plan (2)Lead/Ball FinishMSL Peak Temp (3)no Sb/Br)TL071CPWLE OBSOLETE TSSOP PW 8TBD Call TI Call TITL071ID ACTIVE SOIC D 875Green (RoHS &no Sb/Br)CU NIPDAU Level-1-260C-UNLIM TL071IDE4ACTIVE SOIC D 875Green (RoHS &no Sb/Br)CU NIPDAU Level-1-260C-UNLIM TL071IDR ACTIVE SOIC D 82500Green (RoHS &no Sb/Br)CU NIPDAU Level-1-260C-UNLIM TL071IDRE4ACTIVE SOIC D 82500Green (RoHS &no Sb/Br)CU NIPDAU Level-1-260C-UNLIM TL071IJG OBSOLETE CDIP JG 8TBD Call TI Call TITL071IP ACTIVE PDIP P 850Pb-Free (RoHS)CU NIPDAU Level-NC-NC-NC TL071IPE4ACTIVE PDIP P 850Pb-Free (RoHS)CU NIPDAU Level-NC-NC-NC TL071MFKB OBSOLETE LCCC FK 20TBD Call TI Call TI TL071MJG OBSOLETE CDIP JG 8TBD Call TI Call TI TL071MJGB OBSOLETE CDIP JG 8TBD Call TI Call TITL072ACD ACTIVE SOIC D 875Green (RoHS &no Sb/Br)CU NIPDAU Level-2-260C-1YEAR TL072ACDE4ACTIVE SOIC D 875Green (RoHS &no Sb/Br)CU NIPDAU Level-2-260C-1YEAR TL072ACDR ACTIVE SOIC D 82500Green (RoHS &no Sb/Br)CU NIPDAU Level-2-260C-1YEAR TL072ACDRE4ACTIVE SOIC D 82500Green (RoHS &no Sb/Br)CU NIPDAU Level-2-260C-1YEAR TL072ACJG OBSOLETE CDIP JG 8TBD Call TI Call TITL072ACP ACTIVE PDIP P 850Pb-Free (RoHS)CU NIPDAU Level-NC-NC-NC TL072ACPE4ACTIVE PDIP P 850Pb-Free (RoHS)CU NIPDAU Level-NC-NC-NC TL072ACPSR ACTIVE SO PS 82000Green (RoHS &no Sb/Br)CU NIPDAU Level-1-260C-UNLIM TL072ACPSRE4ACTIVE SO PS 82000Green (RoHS &no Sb/Br)CU NIPDAU Level-1-260C-UNLIM TL072BCD ACTIVE SOIC D 875Green (RoHS &no Sb/Br)CU NIPDAU Level-1-260C-UNLIM TL072BCDE4ACTIVE SOIC D 875Green (RoHS &no Sb/Br)CU NIPDAU Level-1-260C-UNLIM TL072BCDR ACTIVE SOIC D 82500Green (RoHS &no Sb/Br)CU NIPDAU Level-1-260C-UNLIM TL072BCDRE4ACTIVE SOIC D 82500Green (RoHS &no Sb/Br)CU NIPDAU Level-1-260C-UNLIM TL072BCP ACTIVE PDIP P 850Pb-Free (RoHS)CU NIPDAU Level-NC-NC-NC TL072BCPE4ACTIVE PDIP P 850Pb-Free (RoHS)CU NIPDAU Level-NC-NC-NC TL072CD ACTIVE SOIC D 875Green (RoHS &no Sb/Br)CU NIPDAU Level-2-260C-1YEAR TL072CDE4ACTIVESOICD875Green (RoHS &CU NIPDAULevel-2-260C-1YEAR17-Oct-2005OrderableDevice Status (1)PackageType Package Drawing Pins Package Qty Eco Plan (2)Lead/Ball Finish MSL Peak Temp (3)no Sb/Br)TL072CDG4ACTIVE SOICD 875Green (RoHS &no Sb/Br)CU NIPDAU Level-2-260C-1YEAR TL072CDR ACTIVE SOICD 82500Green (RoHS &no Sb/Br)CU NIPDAU Level-2-260C-1YEAR TL072CDRE4ACTIVE SOICD 82500Green (RoHS &no Sb/Br)CU NIPDAU Level-2-260C-1YEAR TL072CDRG4ACTIVE SOICD 82500Green (RoHS &no Sb/Br)CU NIPDAU Level-2-260C-1YEAR TL072CP ACTIVE PDIPP 850Pb-Free (RoHS)CU NIPDAU Level-NC-NC-NC TL072CPE4ACTIVE PDIPP 850Pb-Free (RoHS)CU NIPDAU Level-NC-NC-NC TL072CPSLE OBSOLETE SOPS 8TBD Call TI Call TI TL072CPSR ACTIVE SOPS 82000Green (RoHS &no Sb/Br)CU NIPDAU Level-1-260C-UNLIM TL072CPSRE4ACTIVE SOPS 82000Green (RoHS &no Sb/Br)CU NIPDAU Level-1-260C-UNLIM TL072CPWR ACTIVE TSSOPPW 82000Green (RoHS &no Sb/Br)CU NIPDAU Level-1-260C-UNLIM TL072CPWRE4ACTIVE TSSOPPW 82000Green (RoHS &no Sb/Br)CU NIPDAU Level-1-260C-UNLIM TL072ID ACTIVE SOICD 875Green (RoHS &no Sb/Br)CU NIPDAU Level-2-260C-1YEAR TL072IDE4ACTIVE SOICD 875Green (RoHS &no Sb/Br)CU NIPDAU Level-2-260C-1YEAR TL072IDG4ACTIVE SOICD 875Green (RoHS &no Sb/Br)CU NIPDAU Level-2-260C-1YEAR TL072IDR ACTIVE SOICD 82500Green (RoHS &no Sb/Br)CU NIPDAU Level-2-260C-1YEAR TL072IDRE4ACTIVE SOICD 82500Green (RoHS &no Sb/Br)CU NIPDAU Level-2-260C-1YEAR TL072IDRG4ACTIVE SOICD 82500Green (RoHS &no Sb/Br)CU NIPDAU Level-2-260C-1YEAR TL072IP ACTIVE PDIPP 850Pb-Free (RoHS)CU NIPDAU Level-NC-NC-NC TL072IPE4ACTIVE PDIPP 850Pb-Free (RoHS)CU NIPDAU Level-NC-NC-NC TL072MFKB ACTIVE LCCCFK 201TBD POST-PLATE Level-NC-NC-NC TL072MJG ACTIVE CDIPJG 81TBD A42SNPB Level-NC-NC-NC TL072MJGB ACTIVE CDIPJG 81TBD A42SNPB Level-NC-NC-NC TL072MUB ACTIVE CFPU 101TBD A42SNPB Level-NC-NC-NC TL074ACD ACTIVE SOICD 1450Green (RoHS &no Sb/Br)CU NIPDAU Level-1-260C-UNLIM TL074ACDE4ACTIVE SOICD 1450Green (RoHS &no Sb/Br)CU NIPDAU Level-1-260C-UNLIM TL074ACDR ACTIVE SOICD 142500Green (RoHS &no Sb/Br)CU NIPDAU Level-1-260C-UNLIM TL074ACDRE4ACTIVE SOIC D 142500Green (RoHS &no Sb/Br)CU NIPDAU Level-1-260C-UNLIM 17-Oct-2005OrderableDeviceStatus (1)Package Type Package Drawing Pins Package Qty Eco Plan (2)Lead/Ball Finish MSL Peak Temp (3)TL074ACJOBSOLETE CDIP J 14TBD Call TI Call TI TL074ACNACTIVE PDIP N 1425Pb-Free (RoHS)CU NIPDAU Level-NC-NC-NC TL074ACNE4ACTIVE PDIP N 1425Pb-Free (RoHS)CU NIPDAU Level-NC-NC-NC TL074ACNSRACTIVE SO NS 142000Green (RoHS &no Sb/Br)CU NIPDAU Level-1-260C-UNLIM TL074ACNSRE4ACTIVE SO NS 142000Green (RoHS &no Sb/Br)CU NIPDAU Level-1-260C-UNLIM TL074BCDACTIVE SOIC D 1450Green (RoHS &no Sb/Br)CU NIPDAU Level-1-260C-UNLIM TL074BCDE4ACTIVE SOIC D 1450Green (RoHS &no Sb/Br)CU NIPDAU Level-1-260C-UNLIM TL074BCDRACTIVE SOIC D 142500Green (RoHS &no Sb/Br)CU NIPDAU Level-1-260C-UNLIM TL074BCDRE4ACTIVE SOIC D 142500Green (RoHS &no Sb/Br)CU NIPDAU Level-1-260C-UNLIM TL074BCNACTIVE PDIP N 1425Pb-Free (RoHS)CU NIPDAU Level-NC-NC-NC TL074BCNE4ACTIVE PDIP N 1425Pb-Free (RoHS)CU NIPDAU Level-NC-NC-NC TL074BCNSRACTIVE SO NS 142000Green (RoHS &no Sb/Br)CU NIPDAU Level-1-260C-UNLIM TL074BCNSRE4ACTIVE SO NS 142000Green (RoHS &no Sb/Br)CU NIPDAU Level-1-260C-UNLIM TL074CDACTIVE SOIC D 1450Green (RoHS &no Sb/Br)CU NIPDAU Level-1-260C-UNLIM TL074CDE4ACTIVE SOIC D 1450Green (RoHS &no Sb/Br)CU NIPDAU Level-1-260C-UNLIM TL074CDRACTIVE SOIC D 142500Green (RoHS &no Sb/Br)CU NIPDAU Level-1-260C-UNLIM TL074CDRE4ACTIVE SOIC D 142500Green (RoHS &no Sb/Br)CU NIPDAU Level-1-260C-UNLIM TL074CNACTIVE PDIP N 1425Pb-Free (RoHS)CU NIPDAU Level-NC-NC-NC TL074CNSRACTIVE SO NS 142000Green (RoHS &no Sb/Br)CU NIPDAU Level-1-260C-UNLIM TL074CNSRE4ACTIVE SO NS 142000Green (RoHS &no Sb/Br)CU NIPDAU Level-1-260C-UNLIM TL074CPWACTIVE TSSOP PW 1490Green (RoHS &no Sb/Br)CU NIPDAU Level-1-260C-UNLIM TL074CPWE4ACTIVE TSSOP PW 1490Green (RoHS &no Sb/Br)CU NIPDAU Level-1-260C-UNLIM TL074CPWLEOBSOLETE TSSOP PW 14TBD Call TI Call TI TL074CPWRACTIVE TSSOP PW 142000Green (RoHS &no Sb/Br)CU NIPDAU Level-1-260C-UNLIM TL074CPWRE4ACTIVE TSSOP PW 142000Green (RoHS &no Sb/Br)CU NIPDAU Level-1-260C-UNLIM TL074IDACTIVE SOIC D 1450Green (RoHS &no Sb/Br)CU NIPDAU Level-1-260C-UNLIM TL074IDE4ACTIVE SOIC D 1450Green (RoHS &CU NIPDAU Level-1-260C-UNLIM 17-Oct-2005Orderable Device Status (1)PackageTypePackage Drawing Pins Package Qty Eco Plan (2)Lead/Ball Finish MSL Peak Temp (3)no Sb/Br)TL074IDR ACTIVE SOICD 142500Green (RoHS &no Sb/Br)CU NIPDAU Level-1-260C-UNLIM TL074IDRE4ACTIVE SOICD 142500Green (RoHS &no Sb/Br)CU NIPDAU Level-1-260C-UNLIM TL074IJ OBSOLETE CDIPJ 14TBD Call TI Call TI TL074IN ACTIVE PDIPN 1425Pb-Free (RoHS)CU NIPDAU Level-NC-NC-NC TL074INE4ACTIVE PDIPN 1425Pb-Free (RoHS)CU NIPDAU Level-NC-NC-NC TL074MFK ACTIVE LCCCFK 201TBD POST-PLATE Level-NC-NC-NC TL074MFKB ACTIVE LCCCFK 201TBD POST-PLATE Level-NC-NC-NC TL074MJ ACTIVE CDIPJ 141TBD A42SNPB Level-NC-NC-NC TL074MJB ACTIVE CDIPJ 141TBD A42SNPB Level-NC-NC-NC TL074MWBACTIVE CFP W 141TBD A42SNPB Level-NC-NC-NC (1)The marketing status values are defined as follows:ACTIVE:Product device recommended for new designs.LIFEBUY:TI has announced that the device will be discontinued,and a lifetime-buy period is in effect.NRND:Not recommended for new designs.Device is in production to support existing customers,but TI does not recommend using this part in a new design.PREVIEW:Device has been announced but is not in production.Samples may or may not be available.OBSOLETE:TI has discontinued the production of the device.(2)Eco Plan -The planned eco-friendly classification:Pb-Free (RoHS)or Green (RoHS &no Sb/Br)-please check /productcontent for the latest availability information and additional product content details.TBD:The Pb-Free/Green conversion plan has not been defined.Pb-Free (RoHS):TI's terms "Lead-Free"or "Pb-Free"mean semiconductor products that are compatible with the current RoHS requirements for all 6substances,including the requirement that lead not exceed 0.1%by weight in homogeneous materials.Where designed to be soldered at high temperatures,TI Pb-Free products are suitable for use in specified lead-free processes.Green (RoHS &no Sb/Br):TI defines "Green"to mean Pb-Free (RoHS compatible),and free of Bromine (Br)and Antimony (Sb)based flame retardants (Br or Sb do not exceed 0.1%by weight in homogeneous material)(3)MSL,Peak Temp.--The Moisture Sensitivity Level rating according to the JEDEC industry standard classifications,and peak solder temperature.Important Information and Disclaimer:The information provided on this page represents TI's knowledge and belief as of the date that it is provided.TI bases its knowledge and belief on information provided by third parties,and makes no representation or warranty as to the accuracy of such information.Efforts are underway to better integrate information from third parties.TI has taken and continues to take reasonable steps to provide representative and accurate information but may not have conducted destructive testing or chemical analysis on incoming materials and chemicals.TI and TI suppliers consider certain information to be proprietary,and thus CAS numbers and other limited information may not be available for release.In no event shall TI's liability arising out of such information exceed the total purchase price of the TI part(s)at issue in this document sold by TI to Customer on an annual basis. 17-Oct-2005IMPORTANT NOTICETexas Instruments Incorporated and its subsidiaries (TI) reserve the right to make corrections, modifications, enhancements, improvements, and other changes to its products and services at any time and to discontinue any product or service without notice. Customers should obtain the latest relevant information before placing orders and should verify that such information is current and complete. All products are sold subject to TI’s terms and conditions of sale supplied at the time of order acknowledgment.TI warrants performance of its hardware products to the specifications applicable at the time of sale in accordance with TI’s standard warranty. T esting and other quality control techniques are used to the extent TI deems necessary to support this warranty. Except where mandated by government requirements, testing of all parameters of each product is not necessarily performed.TI assumes no liability for applications assistance or customer product design. Customers are responsible for their products and applications using TI components. T o minimize the risks associated with customer products and applications, customers should provide adequate design and operating safeguards.TI does not warrant or represent that any license, either express or implied, is granted under any TI patent right, copyright, mask work right, or other TI intellectual property right relating to any combination, machine, or process in which TI products or services are used. Information published by TI regarding third-party products or services does not constitute a license from TI to use such products or services or a warranty or endorsement thereof. Use of such information may require a license from a third party under the patents or other intellectual property of the third party, or a license from TI under the patents or other intellectual property of TI.Reproduction of information in TI data books or data sheets is permissible only if reproduction is without alteration and is accompanied by all associated warranties, conditions, limitations, and notices. Reproduction of this information with alteration is an unfair and deceptive business practice. TI is not responsible or liable for such altered documentation.Resale of TI products or services with statements different from or beyond the parameters stated by TI for that product or service voids all express and any implied warranties for the associated TI product or service and is an unfair and deceptive business practice. TI is not responsible or liable for any such statements. Following are URLs where you can obtain information on other Texas Instruments products and application solutions:Products ApplicationsAmplifiers Audio /audioData Converters Automotive /automotiveDSP Broadband /broadbandInterface Digital Control /digitalcontrolLogic Military /militaryPower Mgmt Optical Networking /opticalnetwork Microcontrollers Security /securityTelephony /telephonyVideo & Imaging /videoWireless /wirelessMailing Address:Texas InstrumentsPost Office Box 655303 Dallas, Texas 75265Copyright 2005, Texas Instruments Incorporated。
KEMET PME264系列金属胶电容器产品说明书

Benefits• Approvals: ENEC, UL, cUL• Rated voltage: 660 VAC 50/60 Hz • Capacitance range: 0.001 – 0.1 µF • Lead spacing: 15.2 – 25.4 mm • Capacitance tolerance: ±20%• Climatic category: 40/85/56, IEC 60068–1• Tape and reel in accordance with IEC 60286–2• RoHS Compliant and lead-free terminationsOverviewThe PME264 Series is constructed of multilayer metallized paper encapsulated and impregnated in self-extinguishing material meeting the requirements of UL 94 V–0.ApplicationsTypical applications include worldwide use inelectromagnetic interference suppression in all X2 and across-the-line applications. These capacitors are also for use in high AC and DC voltage applications such as commutator capacitor in converters and ignition circuits.AC Line EMI Suppression and RC NetworksPME264 Series Metallized Impregnated Paper, Class X2, 660 VACLegacy Part Number SystemNew KEMET Part Number SystemClick image above for interactive 3D contentOpen PDF in Adobe Reader for full functionalityOrdering Options Table Benefits cont'd• Operating temperature range of −40˚C to +85˚C• 100% screening factory test at 3,000 VDC• The highest possible safety regarding active and passive flammability• Excellent self-healing properties ensure long life even when subjected to frequent over-voltages • Good resistance to ionization due to impregnated dielectric • High dV/dt capability• The impregnated paper ensures excellent stability and outstanding reliability properties, especially in applications with continuous operationDimensions – MillimetersFRONT VIEW SIDE VIEWPerformance CharacteristicsEnvironmental Test DataApprovalsEnvironmental ComplianceAll KEMET EMI capacitors are RoHS Compliant.Table 1 – Ratings & Part Number Reference(1) Insert ordering code for lead type and packaging. See Ordering Options Table for available options.Soldering ProcessThe implementation of the RoHS directive has resulted in the selection of SnAgCu (SAC) alloys or SnCu alloys as primary solder. This has increased the liquidus temperature from that of 183ºC for SnPb eutectic alloy to 217 – 221ºC for the new alloys. As a result, the heat stress to the components, even in wave soldering, has increased considerably due to higher pre-heat and wave temperatures. Polypropylene capacitors are especially sensitive to heat (the melting point of polypropylene is 160 – 170ºC). Wave soldering can be destructive, especially for mechanically small polypropylene capacitors (with lead spacing of 5 mm to 15 mm), and great care has to be taken during soldering. The recommended solder profiles from KEMET should be used. Please consult KEMET with any questions. In general, the wave soldering curve from IEC Publication 61760-1 Edition 2 serves as a solid guideline for successful soldering. Please see Figure 1.Reflow soldering is not recommended for through-hole film capacitors. Exposing capacitors to a soldering profile in excess of the above the recommended limits may result to degradation or permanent damage to the capacitors.Do not place the polypropylene capacitor through an adhesive curing oven to cure resin for surface mount components. Insert through-hole parts after the curing of surface mount parts. Consult KEMET to discuss the actual temperature profile in the oven, if through-hole components must pass through the adhesive curing process. A maximum two soldering cycles is recommended. Please allow time for the capacitor surface temperature to return to a normal temperature before the second soldering cycle.Manual Soldering Recommendations Following is the recommendation for manual soldering with a soldering iron.The soldering iron tip temperature should be set at 350°C (+10°C maximum) with the soldering duration not to exceed more than 3 seconds.Recommended Soldering TemperatureSoldering time (sec)S o l d e r i n g i r o n b i t t e m p e r a t u r e (d e g C )0501001502002503004080120160200240T e m p e r a t u r e (°C )Time (s)Soldering Process cont'dWave Soldering Recommendations cont'd1. The table indicates the maximum set-up temperature of the soldering processFigure 12. The maximum temperature measured inside the capacitor:Set the temperature so that inside the element the maximum temperature is below the limit:Temperature monitored inside the capacitor.Selective Soldering RecommendationsSelective dip soldering is a variation of reflow soldering. In this method, the printed circuit board with through-holecomponents to be soldered is preheated and transported over the solder bath as in normal flow soldering without touching the solder. When the board is over the bath, it is stopped and pre-designed solder pots are lifted from the bath with molten solder only at the places of the selected components, and pressed against the lower surface of the board to solder the components.The temperature profile for selective soldering is similar to the double wave flow soldering outlined in this document, however, instead of two baths, there is only one bath with a time from 3 to 10 seconds. In selective soldering, the risk ofoverheating is greater than in double wave flow soldering, and great care must be taken so that the parts are not overheated.ConstructionDetailed Cross SectionSelf-ExtinguishingSelf-ExtinguishingResinMetal ContactLayerMetal ContactLayerMarginMetallized Impregnated Paper(First Layer)MarginMetallized Impregnated Paper(Second Layer)Metal Spraying Impregnated Series Design — Multilayer Impregnated Paper Dielectric2 SectionWinding SchemeMarkingBACKFRONTTOPSeriesSelf HealingPackaging QuantitiesLead Taping & Packaging (IEC 60286–2)Taping Specifi cation(1) Maximum cumulative feed hole error, 1 mm per 20 parts.(2) 16.5 mm available on request.(3) Depending on case size.(4) 15 mm available on request.Lead Taping & Packaging (IEC 60286–2) cont'd Ammo Specifi cationsReel Specifi cationsManufacturing Date Code (IEC–60062)KEMET Electronic Corporation Sales Offi cesFor a complete list of our global sales offi ces, please visit /sales.DisclaimerAll product specifi cations, statements, information and data (collectively, the “Information”) in this datasheet are subject to change. The customer is responsible for checking and verifying the extent to which the Information contained in this publication is applicable to an order at the time the order is placed.All Information given herein is believed to be accurate and reliable, but it is presented without guarantee, warranty, or responsibility of any kind, expressed or implied. Statements of suitability for certain applications are based on KEMET Electronics Corporation’s (“KEMET”) knowledge of typical operating conditions for such applications, but are not intended to constitute – and KEMET specifi cally disclaims – any warranty concerning suitability for a specifi c customer application or use. The Information is intended for use only by customers who have the requisite experience and capability to determine the correct products for their application. Any technical advice inferred from this Information or otherwise provided by KEMET with reference to the use of KEMET’s products is given gratis, and KEMET assumes no obligation or liability for the advice given or results obtained.Although KEMET designs and manufactures its products to the most stringent quality and safety standards, given the current state of the art, isolated component failures may still occur. Accordingly, customer applications which require a high degree of reliability or safety should employ suitable designs or other safeguards (such as installation of protective circuitry or redundancies) in order to ensure that the failure of an electrical component does not result in a risk of personal injury or property damage.Although all product–related warnings, cautions and notes must be observed, the customer should not assume that all safety measures are indicted or that other measures may not be required.KEMET is a registered trademark of KEMET Electronics Corporation.。
6-Prime Time
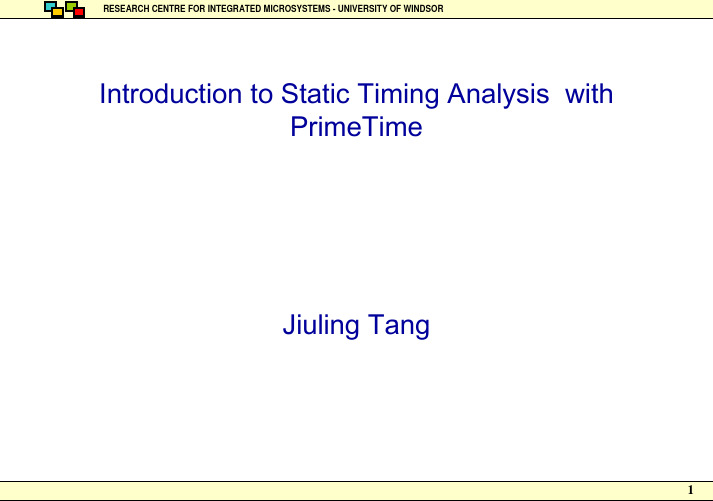
RESEARCH CENTRE FOR INTEGRATED MICROSYSTEMS - UNIVERSITY OF WINDSOR
How STA Works
• •
Find out all valid paths in the design Use technology libraries characterized by SPICE to calculate the delay of each path Check all the path delays to see if setup and hold time have been meet
•
RESEARCH CENTRE FOR INTEGRATED MICROSYSTEMS - UNIVERSITY OF WINDSOR
Timing Paths (continued)
Data1
Logic
D
_ Q
Logic
Data2
D
_ Q
Logic
DataOut1
CLK Clk
ቤተ መጻሕፍቲ ባይዱ
Q
CLK
Q
DataIn2
RESEARCH CENTRE FOR INTEGRATED MICROSYSTEMS - UNIVERSITY OF WINDSOR
Set Up of PT
•
Set up the search_path variable – A list of paths used to locate the design, libraries and other files needed by PT – set search_path {. ./vhdl ./scripts} Set up the link_path variable – Places where PT can find designs and libraries when linking the design – set link_path {* tech_lib.db rams.db} – It is same as DC link_library
模板-使用PrimeTime,PX进行功耗分析
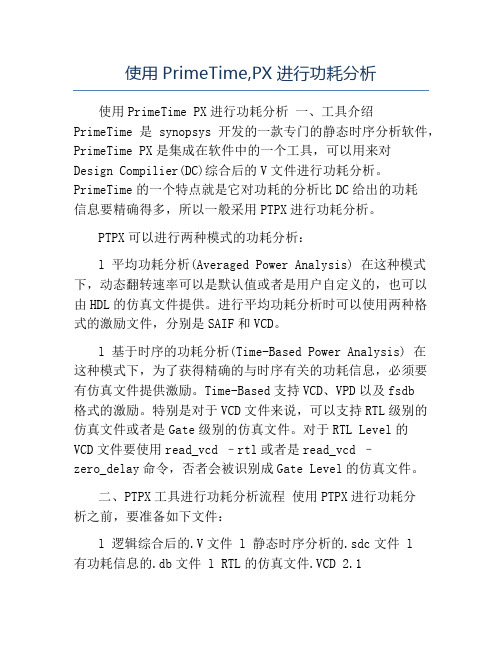
使用PrimeTime,PX进行功耗分析使用PrimeTime PX进行功耗分析一、工具介绍PrimeTime是synopsys开发的一款专门的静态时序分析软件,PrimeTime PX是集成在软件中的一个工具,可以用来对Design Compilier(DC)综合后的V文件进行功耗分析。
PrimeTime的一个特点就是它对功耗的分析比DC给出的功耗信息要精确得多,所以一般采用PTPX进行功耗分析。
PTPX可以进行两种模式的功耗分析:l 平均功耗分析(Averaged Power Analysis) 在这种模式下,动态翻转速率可以是默认值或者是用户自定义的,也可以由HDL的仿真文件提供。
进行平均功耗分析时可以使用两种格式的激励文件,分别是SAIF和VCD。
l 基于时序的功耗分析(Time-Based Power Analysis) 在这种模式下,为了获得精确的与时序有关的功耗信息,必须要有仿真文件提供激励。
Time-Based支持VCD、VPD以及fsdb格式的激励。
特别是对于VCD文件来说,可以支持RTL级别的仿真文件或者是Gate级别的仿真文件。
对于RTL Level的VCD文件要使用read_vcd –rtl或者是read_vcd –zero_delay命令,否者会被识别成Gate Level的仿真文件。
二、PTPX工具进行功耗分析流程使用PTPX进行功耗分析之前,要准备如下文件:l 逻辑综合后的.V文件 l 静态时序分析的.sdc文件 l有功耗信息的.db文件 l RTL的仿真文件.VCD 2.1.V文件、.sdc文件以及.db文件的获得在使用DC完成对工程的综合后,会得到一个如下图所示的V文件(以本实验室综合所用工程rfid2016_v2为例,V文件存放在/rfid2016_v2/flow/syn/result目录下):图2-1 .V文件存放目录 V文件里面的内容如下:图2-2 综合后.V文件内容接下来获取.sdc文件,PTPX用到的.sdc文件和DC用到的.sdc文件一致。
Timing discriminator for pulsed time-of-flight laser rangefinding measurements
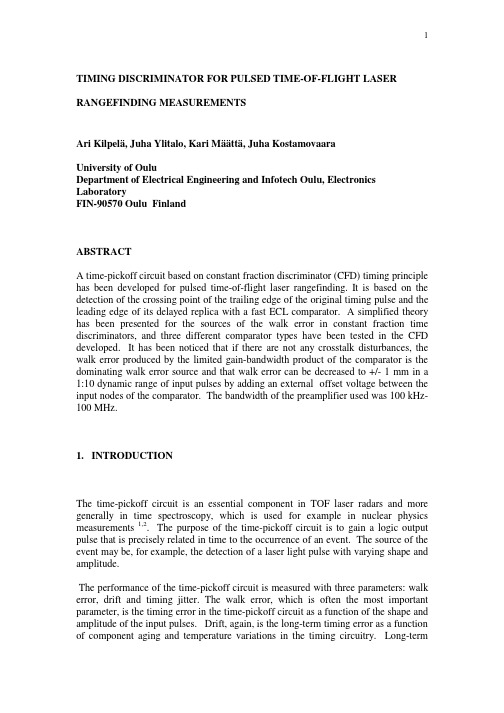
TIMING DISCRIMINATOR FOR PULSED TIME-OF-FLIGHT LASER RANGEFINDING MEASUREMENTSAri Kilpelä, Juha Ylitalo, Kari Määttä, Juha KostamovaaraUniversity of OuluDepartment of Electrical Engineering and Infotech Oulu, Electronics LaboratoryFIN-90570 Oulu FinlandABSTRACTA time-pickoff circuit based on constant fraction discriminator (CFD) timing principle has been developed for pulsed time-of-flight laser rangefinding. It is based on the detection of the crossing point of the trailing edge of the original timing pulse and the leading edge of its delayed replica with a fast ECL comparator. A simplified theory has been presented for the sources of the walk error in constant fraction time discriminators, and three different comparator types have been tested in the CFD developed. It has been noticed that if there are not any crosstalk disturbances, the walk error produced by the limited gain-bandwidth product of the comparator is the dominating walk error source and that walk error can be decreased to +/- 1 mm in a 1:10 dynamic range of input pulses by adding an external offset voltage between the input nodes of the comparator. The bandwidth of the preamplifier used was 100 kHz-100 MHz.1.INTRODUCTIONThe time-pickoff circuit is an essential component in TOF laser radars and more generally in time spectroscopy, which is used for example in nuclear physics measurements 1,2. The purpose of the time-pickoff circuit is to gain a logic output pulse that is precisely related in time to the occurrence of an event. The source of the event may be, for example, the detection of a laser light pulse with varying shape and amplitude.The performance of the time-pickoff circuit is measured with three parameters: walk error, drift and timing jitter. The walk error, which is often the most important parameter, is the timing error in the time-pickoff circuit as a function of the shape and amplitude of the input pulses. Drift, again, is the long-term timing error as a function of component aging and temperature variations in the timing circuitry. Long-termdrift can often be calibrated so that it is sufficiently small. Timing jitter, which isexpressed with the term resolution or precision, is caused by statistical fluctuation ofthe shape of the measurement pulses and noise in the measurement system. The effectof jitter can be reduced by averaging several successive measurement results.In both pulsed laser rangefinding and nuclear physics the amplitude and shape of themeasurement pulse may change in the measurement process. In nuclear physics, theymay vary typically in a relatively large range from one pulse to another due to thedetection process, and, for this reason the gain control cannot be used to cancel theamplitude variation. In pulsed time-of-flight laser rangefinding the variations in thereceived pulse amplitude are induced mostly due to the variation in the measurementdistance and object reflection. The effect of these amplitude variations on timing canusually be cancelled by optical gain control with a negligible timing error. However,the optical gain control is not always fast enough to hold a stable optical peak powerin the pulses. This happens, for example, when the measurement beam is scannedover a surface. In that situation it is necessary to have a time-pickoff circuit with lowwalk error, because the time-pickoff circuit can adapt even to two successive pulses,which have varying amplitudes. It is possible that the averaged shapes of the pulsesmay vary also in the laser rangefinders.The aim of this work was to develop a timing discriminator for pulsed time-of-flightlaser rangefinding measurements with a walk error less than +/-1 mm (+/-7 ps) in aninput amplitude range of 1:5. The application environment is a laser radar describedin chapter 2 and in more detail in ref. 3. It is a double-channel laser radar which isused in steel industry for measuring the thickness of the lining in a steel converter.Tests were made also with a single-channel laser radar, which is designed formeasuring the shapes of ship blocks in shipyards in distances varying from 2 to 30 m 4. In order to decrease the walk error, it was studied how the speed of the timing comparator affects the walk error. Also a compensation method based on theadjustment of the offset voltage of the timing comparator was analyzed. Theadjustment procedure should be easy and fast and applicable to all devices of theselected laser type.2. CONSTRUCTION OF A PULSED TIME-OF-FLIGHT RADARThe block diagram of a double-channel laser radar used in the applicationenvironment in this work is presented in figure 1. The measurement system consistsof a transmitter, two receiver channels, two constant fraction discriminators and a timemeasuring unit. In addition, the system may include also an optical attenuator and amicroprocessor unit which measures the power of the received signal and controls theoperation of the optical attenuator. The start pulses are taken directly from theoutgoing radiation from diffuse reflection. In a single-channel laser radar the wholereceiver channel is common to start and stop pulses, which are separated after thetime-pickoff circuit to their own channels with logic gates. The laser transmitter sendsoptical pulses in 4 kHz frequency. The laser may be either a SH or DH type, the pulse power of which may vary in the range of 5 to 50 W. The FWHM (full-width-at-half-maximum) of the measurement pulses in the channel are in the range of 6 to 8 ns. The lower and upper -3 dB corner frequencies of the whole receiver channel are 100 kHz and 100 MHz, respectively.The time-pickoff circuit converts the analog measurement pulses to ECL logic pulses which are fed to the TDC (time-to-digital converter). The TDC measures the time difference between the start and stop pulses. It consists of a 100 MHz oscillator and counters which roughly digitize the time interval to be measured. The time fractions between the start and stop pulses and their respective next clock pulse but one are digitized with an interpolation circuit based on analog time-to-amplitude conversion 3,5.3. OPERATION OF A TIME-PICKOFF CIRCUIT3.1. GENERAL CONSIDERATIONS OF A TIME-PICKOFF CIRCUITThe time-pickoff circuits can be roughly divided into two groups, constant fraction discriminators and leading edge discriminators. The timing moment may be detected with a comparator 6,7, or a tunnel diode 8. In the leading edge discriminator, the timing moment is the crossing moment of the leading edge of the input pulse and a fixed threshold level. The timing moment is a function of the amplitude and rise time of the input signal. The result of the variation of the timing moment is that the walk error is large and the resolution does not remain at an optimum level.In constant fraction timing, the timing moment is always located at some fractional point of the leading and trailing edges of the input pulse. Usually the input signal is divided into two parts. The other part of the signal is delayed and inverted. An undelayed, but attenuated signal is summed with it. The timing moment is the point where the rising edge of the non-attenuated pulse has an equal voltage level with the peak level of the attenuated pulse. Then the summed bipolar pulse crosses zero voltage level. The optimum fractional point and the value of the delay are selected so that the resolution value is minimized 1. The delay is usually implemented with a delay cable and the attenuation with a resistor divider. Pulse inverting is possible to realize with a pulse transformer or with a shorted delay cable. If a comparator is used in the detection of the timing moment, the delayed and undelayed pulses can be fed to the different input nodes, and subtraction of the pulses from each other is not needed.There are three possible sources for walk error: first, variation of the crossing moment of the two signals in the input of the time-pickoff circuit, second, the limited gain-bandwidth product of the comparator, and third, the possible crosstalk in the circuit.All these sources may increase walk error, but they may also cancel out the effect of each other.The crossing moment may vary due to the timing principle itself or because there is an offset voltage between the two input pulses. The offset voltage may introduce walk error, but it may be also used for cancelling the walk error, which is caused by the limited speed of the comparator. The effect of the offset voltage can be seen from the figure 2, where the input pulse of the time-pickoff circuit is divided into two pulses and the other pulse is delayed. The timing moment is the crossing point of the two pulses which are fed to different input nodes of the comparator. From fig. 2 it can be seen that if a positive offset voltage is added to the delayed pulse and if the amplitude of the input pulse is decreased, the timing moment shifts to an earlier position.The propagation delay of the time-pickoff circuit changes, when the slew rates of the pulses change, because the comparator has a limited gain-bandwidth product. The higher the slew rate of the input pulse, the smaller is the propagation delay of the comparator. If the amplitudes of the input pulses are changed, but the slew rates of the input pulses are kept constant by changing the rise time, the propagation delay remains unchanged, provided that the amplitudes of the input pulses exceed some minimum level 9.The third source for the walk error may be some crosstalk between the input pulses and some other pulses in the time-pickoff circuit. In some cases also the disturbance caused by the crosstalk may cancel the effect of some other walk error source, but then the adjustment procedure of the walk error may be very time-consuming and unpredictable.The timing jitter σt of the measurement result depends on the slew rate and noise of the input pulse:σσt nV D dV dt 222==(/),(1)where σn is the r.m.s. noise of the input of the time-pickoff circuit and (/)dV dt V D =is the slew rate of the signal at the timing moment 10. Eq. 1 is derived for timing,where the input pulse crosses a reference level. From (1) it can be noted that the timing moment should be chosen as a point in which the ratio between noise and slew rate is at minimum. If it is assumed that the distance is measured n times and the noise in the measurement process is randomly distributed, the resolution is improved by factor n compared to a single measurement result.There are many sources of noise in TOF laser radars: the signal-induced noise, the excess noise from an avalanche photodiode and amplifiers and the noise from the current caused by background radiation. According to measurements in ref. 3, when the temperature of the target is near room temperature or lower, the effect of the background noise can be neglected. At small signal levels, both the noise from theelectronics and the signal-induced noise must be taken into account, but at high signal levels the signal-induced noise is dominating, if an avalanche photodiode is used as a photodetector. The higher the timing moment is chosen in the leading or trailing edge, the stronger the signal-induced noise is at the timing moment. At the top of the laser output pulse the slew rate is small and, for example, the relaxation oscillations may cause instability in the shape of the pulse 11. For these reasons the timing moment should not be chosen near the peak of the pulse. The lower the timing moment is at the linear part of the leading or the trailing edges, the better the resolution, because the signal-induced noise is there at its lowest, assuming that the timing moment is chosen from the linear parts of the leading and trailing edges.3.2. CONSTRUCTION OF THE DEVELOPED CFDThe main principle of the time-pickoff circuit used here is the following: the amplified input pulse is divided into two parts which have equal amplitudes and the other pulse is delayed. The delayed and non-delayed signals are fed to the different input nodes of a fast comparator with ECL logic outputs. The value of the delay is selected so that the two pulses cross each other at the trailing edge of the first pulse and at the leading edge of the latter pulse. The pulse diagram is presented in figure 2. With this method, the advantage is that the resolution is small, because both pulses to the inputs have high slew rates at the timing point. The timing moment is usually selected to be at 30 % - 40 % from the maximum amplitude, because, according to the measurements, the resolution is smallest at that level.A simplified schematic diagram of the CFD developed is presented in figure 3. The threshold level of the noise comparator is adjusted to exceed the peak level of the background noise, for example to a level of 100 mV to 150 mV. The output pulse of the noise comparator goes through ECL-flip-flop n:o 1 (type 10H131) to the data input of the flip-flop N2. So the output of the flip-flop N2, which is at the same time the output of the discriminator, can change its state only when the amplitude of the input pulse of the discriminator exceeds the threshold level adjusted to the noise comparator. The output timing pulse from the timing comparator triggers the clock input of flip-flop N2.Flip-flop N1 has two purposes: it extends the data input pulse of the flip-flop N2 and latches the output nodes of the noise comparator to a stable state until the output of the flip-flop N2 resets the state of flip-flop N1. The latter function is important, because the output nodes of the noise comparator may change at the same time as the input pulses to the timing comparator cross each other. Then the output pulse of the noise comparator can be coupled to the input nodes of the timing comparator through the parasitical capacitances between the bonding wires in a dual comparator circuit inducing timing error.The presented configuration is a result of evolution. Originally the timing comparator was enabled by the noise comparator through its latch input only for the period oftiming. The idea was that in this case the offset of the timing comparator can be selected freely without oscillations which may be present in the version of fig. 3 if the noise level exceeds the input offset 5. However, this method needs an extra delay cable in order to adjust the timing for the latch input of the timing comparator. We found that that the latching of the timing comparator is not needed, because the possible oscillations in the output of the timing comparator in the construction of fig.3 are suppressed when the timing pulse exists at its input, and were not found to produce any measurable errors.4. MEASUREMENTSThree different comparator types were tested in a CFD circuit described in chapter 3. The types were Analog Devices AD 96687 and Signal Processing Technology HCMP 96870A and SPT 9689. The walk error of the distance meter was measured with several offset voltages with all three comparator types. All the comparators tested are pin-compatible with each other. The main difference between the comparators is in the -3 dB bandwidth of small signal open loop gain. However, not any exact values are given in the datasheets, but it seems that the SPT9689 is the fastest comparator and the AD96687 is the slowest one.In all walk error measurements a double-channel laser radar was used, except for the measurement presented in figure 8, which was measured with a single-channel laser radar. In the single-channel radar only the comparator SPT9689 was tested. The semiconductor laser used in double-channel radar was a pigtailed DH laser diode CVD-193F (manufactured by Laser Diode Inc.), the pulse power of which was 18 W measured from the end of the fiber. The rise and fall times (between 10 % and 90 %) of the channel pulse (at the input of the CFD) were 4.5 ns and 4.7 ns, respectively, and the FWHM was 7.9 ns measured with an oscilloscope of 1 GHz analog bandwidth. The shape of the channel pulse is presented in figure 4. In a single-channel laser radar the laser type CVD-93F was used. It had a pulse power of 5 W, rise time of 3.3 ns, fall time of 4.3 ns and FWHM of 6.8 ns. The delay cable in the constant fraction discriminator was 190 cm long in the measurements presented in figures 5 to 12. With that cable the crossing point of the undelayed and delayed pulses was about at 41 % of the maximum height of the pulse in a double-channel radar and 28 % in the single-channel radar.The walk error measurements with zero offset voltage are presented in figure 5. The external offset voltage compensated the internal offset voltage of the comparator. We found that the walk error was clearly largest with the AD 96687 comparator and smallest with the SPT 9689 comparator.The walk error measurements with different offset voltages are presented in figures 6 to 9. For all comparators the walk error curves have been measured with several values of offset voltage. The smallest walk error, +/- 1 mm in the input amplitude range of 0.2 to 2.0 V, was achieved with SPT 9689 in a double-channel radar. The best measured walk error in the single-channel radar with SPT9689 was about +/- 1.5 mm in the input amplitude range of 0.2 to 2.0 V.The effect of the timing level on the performance of the CFD was studied by measuring the single-shot resolution values vs. input pulse amplitudes with several delay cable lengths (figure 10). The comparator type was AD96687. The timing levels are indicated as relative levels of the crossing point of the input pulses of the timing comparator compared to the maximum amplitude of the pulses. The timing levels were measured with zero offset voltage. The results show that the resolution depends slightly on the timing level, as expected, and the higher the timing level, the worse the resolution. However, with input amplitudes smaller than 1.3 V the resolution was clearly the best with 41 % timing level and not with the lowest timing level. This is probably due to the fact that with low signal amplitudes, the noise of the amplifiers becomes the dominating noise source and the total noise levels at the timing points at 23 % and 41 % timing levels are somewhat equal. The lower slew rate value at 23 % level gives a worse resolution than at 41 % level at low signal amplitudes.The effect of crosstalk between the output of the noise comparator and the input of the timing comparator may have an impact on the shape of the walk error curve. It was proved to be true by measuring the walk error curves so that the latch inputs of the noise comparator were not in use. The walk error curves measured with and without using the latch inputs, with different noise comparator threshold values, are presented in figures 11 and 12, respectively. In both measurements the comparator type was AD96687. All curves in the figures are measured with the same delay cable length and the same timing comparator offset voltage. It can be seen that the crosstalk between bonding wires inside the double comparator circuit has a rather strong effect on the shape of the walk error curves and for this reason it is necessary to prevent the change of state of the output nodes of the noise comparator during the timing moment at the inputs of the timing comparator.5. EVALUATION OF THE WALK ERROR VALUEIn the evaluation of the value of the walk error, two things must be taken into account: the dependence of the propagation delay on the slew rate of the input pulses and the offset voltage in the input nodes of the comparator.A simplified model of the propagation delay of a comparator has been introduced in12. The propagation delay is created by the internal capacitances of the comparator. In the following, we make a simple assumption that the comparator is an amplifier which has a single high-frequency pole and the shape of the input pulses remains the same at all input signal amplitudes. The amplification is linear, but we take into account the effect of operating in a large signal range by including in the calculation the minimum propagation delay τD. It is assumed that the maximum slew rate of the comparator is not exceeded. The open loop gain in the s-domain is:U s U s F se AsAo is D()()()()()==⋅+−ττ10(2),where A(0) is the open loop gain in zero frequency and τ001= the time constant corresponding to the upper -3 dB corner frequency of the unit gain. The terms U i(s) and U o(s) are the input and output voltages of the amplifier, respectively. Assuming that the input signal is a linear ramp ()u t SR ti=⋅and taking the inverse Laplace transform from U o(s) we can calculate the response time t delay required to reach some output level V o:tVSRdelay D=⋅+200ττ(3)Eq. (3) consists of the minimum propagation delay τD and another delay, the value of which depends on the slew rate of the input signal. The propagation delay has the simplified form in small signal analysis:t AB slew ratedelay=+,(4)where A ja B are constants. The smaller is the constant 200⋅Vτ, the smaller the dispersion of the propagation delay (walk error) of the comparator. In practice it means that the comparator should be as fast as possible.The effect of the offset voltage summed to the input nodes of the comparator can be evaluated coarsely using input pulses which have linear leading and trailing edges (figure 13). Now we derive the crossing point of the pulses from a pair of equations which define the straight lines of the leading and trailing edges of the pulse:u U U t t u U U t t of pr p pf−=⋅−=−⋅(5),where U of = offset voltage between the input nodes, U p = the amplitude of the input pulses in the comparator nodes, t r = rise time (0-100 %) of the pulse and t f = fall time (100%-0) of the pulse. We can calculate the crossing point t cross by solving the pair of equations in the crossing point:t U U t t cross of pr f =−+111(6)If U of , t r ja t f are constants, we come to a simplified solution for the crossing point:t C D slew ratecross =− (7),where C and D are constants and the term slew rate equals to the sum of the slew rates of the leading and trailing edges: slew rate U t U t p r p f=+.In order to calculate the final walk error curves, we may select the values V 0 and τ0 in eq. 3 so that the shape of the calculated propagation delay curve is close to the shape of the measured propagation delay curve of AD96687 presented in figure 5. For example, if we choose the value of 0.5 V for V 0 and assume that the AD96687 has a gain of 170 at the frequency of 100 MHz, the calculated walk error curve comes very close to the measured one. If we sum up equations 3 and 6 and use the measured rise and fall times t r and t f , we can calculate the walk error curves with different offset voltage values. The result of such calculations for AD96687 is presented in figure 14.In equation 6 the rise time t r (0-100 %) used was 4.9 ns and the fall time t f (100%-0)used was 5.6 ns, and in equation 3 the rise and fall times used were 5.6 ns both.Comparing the calculated walk error curves of figure 14 and the measured walk error curves of figure 6 with each other, it seems that they are reasonably close to each other and it can be concluded that the forming of the walk error curve is based on the factors described above. The value of the walk error can be decreased in a limited amplitude range by adding an external positive voltage between the input nodes of the timing comparator, as the calculations show. The differences between calculated and measured curves are probably due to the facts that the comparator propably has more than one high-frequency poles and that the pulse edges in equation 5 have been approximated to be linear, which in reality is not, of course, quite true.6. DISCUSSIONTo achieve a small walk error, the bandwidth of the timing comparator should be increased. In this way only a small offset voltage is needed to straighten the walk error curve and the result is a decreased walk error value especially at low amplitudes. From eq. 5 it can also be seen that if the rise and fall times of the input pulses are decreased, the walk error decreases.The new semiconductor technologies make it possible to fabricate faster amplifiers and comparators. There are already commercially available transimpedance and voltage amplifiers with bandwidths ranging from 0.5 to 1 GHz. However, with high bandwidths, the importance of the layout increases and all stray capacitances must be minimized in order to prevent other parts of the circuit from disturbing the input pulses of the timing comparator. The crosstalk could also be minimized by integrating the CFD with the amplifiers on the same semiconductor chip. ACKNOWLEDGMENTSThe authors would like to thank the Academy of Finland for financial support of the work.REFERENCES1 D.A. Gedcke, W.J. McDonald, Nuclear Instruments and Methods, 58, 253 (1968)2 T.J. Paulus, IEEE Transactions on Nuclear Science, 3, (1985)3 Kari Määttä, Juha Kostamovaara, Risto Myllylä Applied Optics, 27, 5334 (1993)4 Kaisto I., Kostamovaara J., Manninen M., Myllylä R., SPIE Proceedings 2088, 121 (1993)5 Juha Kostamovaara, Kari Määttä, Risto Myllylä, SPIE Proceedings 1614, 283 (1991)6 Michael R. Maier, Donald A. Landis, Nuclear Instruments and Methods, 117, 245, (1974)7 M.D. Cable, M.S. Derzon, R.G. Vieira, H.P. Spracklen, IEEE Transactions on Nuclear Science, 35(1), 133, (1988)8 Branko Leskovar, C.C. Lo, Paul R. Hartig, Kenneth Sauer, Rev. Sci. Instrum., 47(9), 1113, (1976)9 David M. Binkley, Michael E. Casey, IEEE Transactions on Nuclear Science, 35(1), 226, (1988)10 Bertolini, G., Coche, A., Semiconductor detectors, , pp. 243-276, North-Holland Publishing Co., Amsterdam (1968)11 Peter K. Cheo: "Handbook of Solid-State Lasers", pp. 82-84, ISBN 0-8247-7857-X, Marcel Dekker Inc., New York (1989)12 Arie F. Arbel, "Analog Signal Processing and Instrumentation", pp.172-176, Cambridge University Press (1980)Figure 1. A simplified diagram of a laser radar.Figure 2. The formation of timing point, when an offset voltage has been added between the input nodes of the timing comparator.Figure 3. The schematic diagram of a constant fraction time discriminator used in this work.Time [ns]C h a n n e l p u l s e [V ]00.20.40.60.811.2010203040Figure 4. The delayed and non-delayed pulses measured from the input pins of the comparator AD96687. The offset voltage between the input pins was 36 mV and the timing level was 41 %.Figure 5. The walk error of the CFD for three comparator types with zero external offset voltage and 41 % timing level.offset voltages. The timing level was 41 %.Figure 7. The measured walk error curves of the CFD with HCMP96870A and different offset voltages. The timing level was 41 %.Figure 8. The measured walk error curves of the CFD with SPT 9689 and different offset voltages. The timing level was 41 %.Figure 9. The measured walk error curves of SPT 9689 with different offset voltages in single-channel laser radar. The timing level was 41 %.Figure 10. The single-shot resolution of the laser radar as a function of the amplitude of the channel pulse measured with four different timing levels. The comparator type was AD96687 and the offset voltage was 33 mV.Figure 12. The walk error curves measured with different noise comparator threshold values without using the latch inputs of the noise comparator. The timing level was 41 % and the offset voltage of the timing comparator was 33 mV.。
Class2_15_16_Peak_Distortion_Analysis
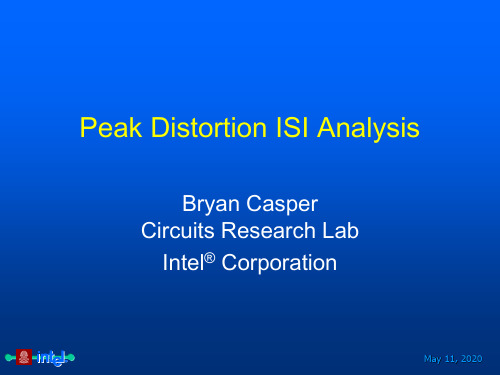
Peak Distortion Analysis
Bryan Casper - CRL
August 11, 2013
LTI property: Superposition of coupled symbols
Out
Insertion loss response
Tx symbol …000010011100…
Bryan Casper - CRL
August 11, 2013
Worst-case 1
0101100000
VWC1 cursor ISI
Peak Distortion Analysis
Casper - CRL
August 11, 2013
负值:1 1 正值:0 0
0 1
Tx symbol (mirror)
Impulse response
Pulse response
Peak Distortion Analysis
Bryan Casper - CRL
August 11, 2013
LTI property: Superposition
In Out
Tx symbol
VWC0 VWC1 2
ISI cursor ISI
Tx symbol …000010011100…
Composite response
Peak Distortion Analysis
Bryan Casper - CRL
August 11, 2013
Max data rate calculation method
• Determine maximum value of all sample timing uncertainty (not including ISI)
1、kick-off presentation
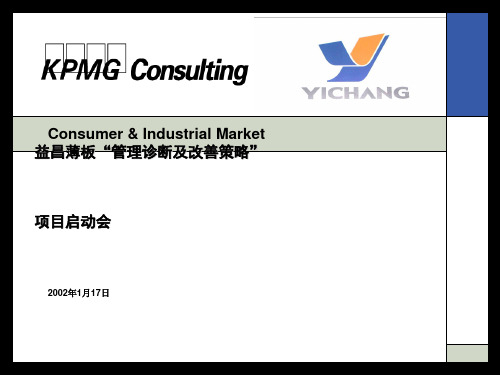
Emerging - Vision
Hub for interaction, of structured & unstructured data: discussion DB, groupware apps, real-time info feed, etc.
Move knowledge management collaboration efforts toward Electronic Process Interchange (EPI) and collaborative exchange platforms:
Focus the knowledge at the businessprocess-level (vs. data level)
Conduct a greater level of info/knowledgesharing through common business planning and everyday processes
该节包括: 1 2 3
© 2002 KPMG Consulting Inc. All rights reserved. Page 9
流程1最佳流程汇总
样例
Consumer & Industrial Markets
A. 在整个公司内发展一套企业化的知识管理战略。 B. 将知识管理战略和公司的其他业务战略和目标相整合。 C.将知识管理战略和公司的IT战略和架构相整合(包括业务数据库,安全和数据等等)。 D. 通过标准的变化管理将知识管理战略流程建立在组织的结构的流程中。 F. 在组织内多个试点推广知识管理。 G. 允许员工创建知识管理。
益昌同样面对着国内外薄板生产企业的竞争。根据薄板行业的特点,其利润空间局限于热轧原板和 冷轧薄板的价差,而热轧原板的成本和冷轧薄板的售价完全处于市场的调控下。在产品、技术、人 才方面,益昌已经和国际先进水平实现了接轨,通过在企业内部挖潜力,通过提高管理水平,降低 成本是益昌面临的重要挑战,也是益昌酝酿已久的课题。
PAMSTAnP2G_V2007-cin

I63
I22 v2005.0 V2007.0 Speed up 493 441 1.12
I30 6828 4540 1.50
I63 1494 322 4.64
I22 I30
25
Amélioration du temps de découpe 2/2
Maillage Flan v2005 Contour flan Maillage Flan v2007
Anne CHAMBARD Yann STRUB Pierre MARQUETTE - 07/02/2007 -
PAMSTAMP2G V2007.0 Présentation des nouveautés
de l’interface graphique pour le module AutoStamp
23
Transformation maillage 3/3
-Ajout d’une découpe Amélioration de l’algorithme de découpe v2005.0 v2007.0
24
Amélioration du temps de découpe 1/2
SMP-DP 1 proc avec Linux amd64
MODES: Part preparation Blankholder Addendum Simulation model Reverse Engineering
3
Nouvelle fonctionnalité: Auto-Addendum
Création automatique de profils autour de la pièce 2 types de profiles: l’un est sélectionné par l’utilisateur, l’autre est automatique de type spline (angle mur pièce>30° avec la direction d’emboutissage)
COPC Bohai ISO Training Presentation Rev 1 10-30-2007
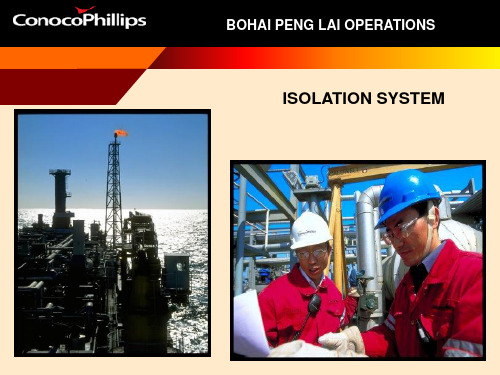
Bohai Operations
NEW DEFINITIONS FOR TEAM ROLES & ISOLATION CONCEPTS Isolation Definitions Authorizers, Responsible Persons, Issuers, and Work Leaders are the same employees as identified through the PTW procedure.
Bohai Operations Work Leader
The Work Leader ensures: •Safe conditions at the worksite shall be maintained at all times. •All Permit Users in the workgroup are briefed according to PTW requirements. •All Permit Users in the workgroup are given an opportunity to personally inspect and verify energy isolations if they so wish.
Bohai Operations Authorized Person
The Authorized Person reviews the Isolation Certificate and EIP, and ensures that all safety and conflicting work considerations have been addressed, and all supporting documentation such as P&IDs are attached to the permit package.
TL9000测量指标培训教材参考资料
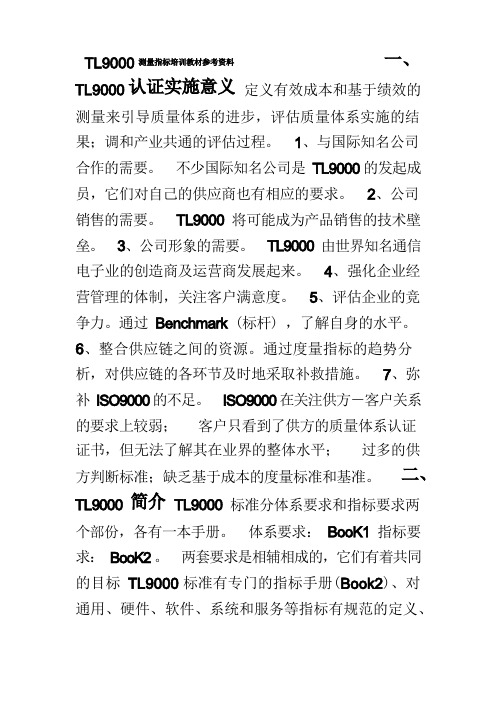
定义有效成本和基于绩效的测量来引导质量体系的进步,评估质量体系实施的结果;调和产业共通的评估过程。
、与国际知名公司合作的需要。
不少国际知名公司是的发起成员,它们对自己的供应商也有相应的要求。
销售的需要。
将可能成为产品销售的技术壁垒。
由世界知名通信电子业的创造商及运营商发展起来。
营管理的体制,关注客户满意度。
争力。
通过(标杆) ,了解自身的水平。
析,对供应链的各环节及时地采取补救措施。
补的不足。
在关注供方-客户关系的要求上较弱;客户只看到了供方的质量体系认证证书,但无法了解其在业界的整体水平;过多的供方判断标准;缺乏基于成本的度量标准和基准。
标准分体系要求和指标要求两个部份,各有一本手册。
体系要求:指标要求:。
两套要求是相辅相成的,它们有着共同的目标标准有专门的指标手册、对通用、硬件、软件、系统和服务等指标有规范的定义、采集原则、计算公式和报告模板。
根据的规定,所有申请认证企业须必须按指标手册规范建立衡量指标体系,并分季度向授权的数据管理中心(美国德州大学)报送企业每月的衡量指标报告,并获得数据有效的确认函;指标的意义、提供业界做为标杆的指引——内部质量改进—与客户关系在正常运输、装卸操作中,由于非正常装运条件造成的FRU 损坏,但包装FRU 的包装箱或者容器并没有损坏的情况下,这种情况下退回的FRU 仍作为退货,计入退货总数中;所有退货应以FRU 为单位计数,如实际退回的产品组件中包含多个FRU 时,则退货数以实际退回的FRU 数计算。
2、以下情况不计入退货总数计算:按计划召回、轮换,且在产品更换前并未发生故障的FRU ;由于车祸、漏水、电信号峰值超过规定限制、或者其他超过设备设计使用条件的因素造成损坏的FRU ;因合同问题原因(包括合同的错误或者合同中故意的额外采购)而非产品本身问题造成交付后返回的FRU ;所有公司内部实验环境、展览情况返回的FRU 。
Figure 5 Equivalent currents . a J and .

Ž.Ž.Figure 5Equivalent currents a J and b M on a dielectric x y Ž.⑀s 3.0cube of 0.5m side length at x s 0,y s 0.125m,z s r 0.25m illuminated by a Gaussian plane wave.Number of edges s72Ž.Figure 6Backscattered s 0Њ,s 0Њfar-field response of a Ž.dielectric ⑀s 3.0cube of 0.5m side length illuminated by a r Gaussian plane wave.Number of edges s 72present the IDFT solution obtained by solving the same problem in the frequency domain at 128samples in the range 0᎐400MHz and inverse transformed into the time domain.We note a good comparison between both solutions.Ž.Next,we consider a 0.5m dielectric cube ⑀s 3.0cen-r tered at the origin.Each face of the cube is divided into eight triangular patches with a total number of 72edges and 144unknowns.Again,the time step for this problem is 0.25LM.Figures 5and 6show the equivalent currents and the back-scattered far-zone electric field,respectively,obtained using the implicit solution scheme.Note that the equivalent cur-rents are sampled on the top face of the cube at x s 0m and y s 0.125m.For comparison,we also present the IDFT solution.A good agreement in both results is evident for this example stly,we note the absence of any trace of instabilities in both of the examples presented.5.CONCLUSIONSIn this work,we have presented a computationally efficient method to the dielectric body problem by solving a set of coupled time-domain integral equations.The solution methodpresented in this work is simple,accurate,and free of late-time instabilities.REFERENCES1.D.A.Vechinski,S.M.Rao,and T.K.Sarkar,Transient scattering from three-dimensional arbitrarily shaped dielectric bodies,J Opt Ž.Soc Amer 111994,1458᎐1470.2.B.P.Rynne,Time domain scattering from dielectric bodies,Elec-Ž.tromagnetics 141994,181᎐193.3.S.M.Rao and T.K.Sarkar,Time domain modeling of two dimen-sional conducting cylinders utilizing an implicit scheme,Mi-Ž.crowave Opt Technol Lett 151997,342᎐347.4.S.M.Rao and T.K.Sarkar,Transient analysis of electromagnetic scattering from wire structures utilizing an implicit time domain integral equation technique,Microwave Opt Technol Lett 16Ž.1998,66᎐69.5.S.M.Rao and T.K.Sarkar,An efficient method to evaluate the time-domain scattering from arbitrarily shaped conducting bodies,Ž.Microwave Opt Technol Lett 171998,321᎐325.6.A.A.Kishk and L.Shafai,Different formulations for numerical solution of single and multi-bodies of revolution with mixed boundary conditions,IEEE Trans Antennas Propagat AP-34Ž.1986,666᎐673.7.D.A.Vechinski and S.M.Rao,Transient scattering from dielectric cylinders ᎏE-field,H-field,and combined field solutions,Radio Ž.Sci 271992,611᎐622.8.R.F.Harrington,Field computation by moment methods,Macmil-lan,New York,1968.9.D.R.Wilton,S.M.Rao, A.W.Glisson, D.H.Schaubert,O.M.Al-Bundak,and C.M.Butler,Potential integrals for uniform and linear source distributions on polygonal and polyhedral domains,Ž.IEEE Trans Antennas Propagat AP-321984,276᎐281.ᮊ1999John Wiley &Sons,C 0895-2477r 99MODELING OF NOLMDEMULTIPLEXERS EMPLOYINGOPTICAL SOLITON CONTROL PULSEZ.Ghassemlooy,1C.Y.Cheung,1and A.K.Ray 11Electronics Research Group School of Engineering Sheffield Hallam University Sheffield S11WB,England Recei ¨ed 7October 1998ABSTRACT:An optical soliton pulse may be used as a control signal in nonlinear optical loop mirror demultiplexers in order to reduce timing-jitter noise and crosstalk.A mathematical model for calculating the width of the soliton switching window and optimizing critical parameters such as walk-off time and pulse width has been presented,and its accuracy is ¨erified by sol ¨ing the nonlinear Schrodinger equation.ᮊ1999John Wiley &Sons,Inc.Microwave Opt Technol Lett 21:205᎐208,1999.Key words:semiconductor laser amplifier;optical switching;soliton;optical time-di ¨ision demultiplexer;optical loop mirrors 1.INTRODUCTIONŽ.The nonlinear optical loop mirror NOLM demultiplexer is a promising configuration for achieving all-optical time-division w x demultiplexing because of its high operating speed 1.Chan-nel demultiplexing is realized by the phase difference be-Ž.Ž.tween the clockwise CW and counterclockwise CCW sig-nal pulses propagating within the fiber loop;see Figure 1.In .ultra-high-speed systems,there are two major problems:1difficulty in achieving complete switching of the signal pulses,.and 2timing jitter between the copropagating control and signal pulses.The latter is reduced to a certain extent by the introduction of walk-off time T between the control and w signal pulses.This results in a square switching window shape,with a width of T L ,where L is the fiber loop length,w rather than a bell shape,thus enabling improved switching,w x and consequently,better tolerance to timing jitter 2.A 100%switching is difficult to achieve because of the control pulse experiencing a soliton compression effect due to the interaction between the fiber dispersion and self-phase modu-Ž.w x lation SPM 4.This will result in an increased control signal peak power,which in turn leads to an asymmetrical switching window with an increasing phase shift toward the end of the switching profile.Maximum switching may be achieved by employing an optical soliton as a signal or control pulse since the fundamental properties of the soliton are uniform phase over the entire pulse and constant pulse shape over the entire propagation length.Here,we investi-gate the latter option,and show that the problem of pulse deformation during propagation within the loop is solved,thus resulting in a much improved switching window with high transmittance and reduced timing jitter effects.2.THEORYTo study the output transmittance of the NOLM demulti-plexer,it is best to consider the combined impact of the .Ž..following effects:1cross phase modulation XPM ,2propa-.gation of optical soliton pulse within the fiber loop,and 3walk-off time between the control and signal pulses.The switching window is created by the phase difference between the CW and CCW signals.The phase of the signal pulse copropagating with the control pulse is substantially changed by the XPM effect.With the inclusion of walk-off time,the phase change can be represented byLŽ.Ž.⌬s 2␥P T y T иx dx1H w 0where ␥is the nonlinear coefficient,P is the optical power profile,and T is the walk-off time per unit length.w The control pulse has to meet the following soliton condi-tions in order to propagate undistortedly along alosslessFigure 1Typical configuration of NOLM demultiplexerw x fiber 6:1.the propagation equation of a fundamental soliton wave Ž.Ž.Ž.u ,s sech exp i r 2<<22.the peak power P s r ␥T 020where u is the normalized amplitude,s T r T and s o z r L ,z is the traveling distance,L is the dispersion length D D 2<<s T r ,T f FWHM r 1.763is the pulse width of the o 20optical soliton control pulse,and is the first-order disper-2sion coefficient.The control pulse walks through the signal pulse due to the difference in the group velocity,and with the phase change of the signal depending on the value of the total walk-off time,the time-varying optical power profile in Ž.Eq.1can be replaced by the pulse average power over the duration of total walk-off time.The maximum average power of the control pulse,resulting in XPM,is given asT r 2t w Ž.P T dTH y Tr 2t w Ž.P s.2ave Žmax .T t wŽ.<<2For a soliton pulse,P T s P иu ;therefore,the peak 0phase change can be shown to be T r 2T t w 02Ž.Ž.Ž.⌬s 2␥LP T sech T r T d T r T T 3H p 0000t w y Tr 2T t w 0where T s T L is the total walk-off time.t w w Substituting for P and replacing ⌬by for maximum 0p 2Ž.transmittance and solving for sech x ,we obtain an expres-sion for the lossless fiber loop as<<Ž.Ž.T T s 4L tanh T r 2T .40t w 2t w 0The output transmittance would be equal to 1if the condi-Ž.Ž.tion set by Eq.4is satisfied.In Eq.4,it is assumed that the fiber loop is loss free.However,in NOLM demultiplexers,where a long length of fiber is used,it is necessary to include the fiber loss ␣in the analysis.Assuming that the rate of decrease of power is so small,the increase in chromatic dispersion can be neglected,and therefore the peak power can be expressed as1LŽ.Ž.Ž.P sP 0exp y ␣z dz .5H 00L 0Ž.Ž.Solving Eq.5and substituting it into Eq.3,we can obtaina modified expression for maximum transmittance as<<w Ž.x Ž.Ž.T T ␣s 41y exp y ␣L tanh T r 2T .60t w 2t w 0The output transmittance would be equal to 1if the condi-Ž.tion set by Eq.4is satisfied.In NOLM employing a Gauss-ian pulse,the switching window profile is a bell shape with a nonflat top.Therefore,the relative timing jitter between the control and signal pulses and the control pulse deformation within the loop will induce intensity fluctuation of the demul-tiplexed output signal,thus resulting in a switching power w x penalty 5,whereas with an optical soliton pulse,the shape of the switching window becomes symmetrical with a flat-top transmittance profile,thus resulting in reduced timing jitter noise and interchannel crosstalk.To achieve the former,theFigure 2Typical soliton transmission window:the shaded areas represent the portion of the control pulse overlapping with the signal pulse throughout the complete propagationvariation of transmittance at the top of the switching window has to be kept within the perturbation-tolerance factor F ;p see Figure 2.The shaded areas represent the portion of the control pulse profile walking through the signal pulse along the whole propagating distance.At time t ,the transmittance 1level is below the threshold level.From t to t ,it remains 13above the threshold level,and reaches peak value at time t .2After t ,the transmittance level begins to drop.Therefore,3the width of the transmission window is equal to t y t .By 31comparing the walk-off region of the pulse profile at t ,t ,12and t ,the walk-off region of the pulse profile at t is just left 31Ž.shifted with t s T r 2y T and t is just right shifted with t w 13Ž.t s T y T r 2from the symmetric walk-off region of the 2t w Ž.Ž.pulse profile at t ,respectively.T y T r 2s T r 2y T 22t w t w 1because of the symmetrical shape of a soliton pulse.The transmission window’s perturbation-tolerance width is de-fined as the period of time at which the normalized transmit-tance level remains above the threshold value 1y F ,which p is given asŽ.Ž.<Ž.<Ž.W s 2T r 2y T s 2T y T r 2s 2T r 2y T 7t w 12t w t w i where T and T are referred to in Figure 2.For F s 0.5,12p W s FWHM width.A model was developed for calculating the width of a soliton switching window assuming that ␣s 0.For a given set of system parameters,F can be related to T p 1and T by the following equation:2y 1Ž.4cos F r tanh Tr 2T 'pt w 0ž/ž/Ž.w Ž.x Ž.s tanh T r T y tanh T y T r T .8i 0i t w 0Ž.Equation 8yields two solutions for T :one is T ,and the i 1other is for T .W can then be calculated by substituting 2Ž.Ž.either of the solutions for T in Eq.8into Eq.7.The i expression for W holds true no matter which one of the solutions of T is used in the substitution.i 3.SIMULATION RESULTS AND DISCUSSIONŽ.Equation 4was solved numerically by the Newton ᎐Raphson Ž.w x method NRM 3,and the solution was checked by applying Ž.the corresponding values of T and T to Eq.4for 0t w verification.For L s 3km,the results for the soliton pulse width versus the total walk-off time for different values of 2and ␣for a soliton switching window at maximum transmit-tance are shown in Figure 3.The soliton pulse width de-creases with the increase of the total walk-off time.This is due to the decrease in the interactive time for XPM between signal pulses and the central peak of control pulses.The reduced pulse width compresses more optical power around the central peak of the control pulses,and as a result,the phase change ⌬is increased.The increased phase change due to reduced values of the soliton pulse width is exactly equal to the decreased phase change due to the larger value of the total walk-off time,thus maintaining maximumtrans-Figure 3Soliton pulse width versus total walk-off time for different values of and ␣2Figure4Perturbation-tolerance width versus total walk-off timefor different values of2mittance.Figure3also shows the effects of thefirst-order dispersion coefficienton the soliton pulse width and the2total walk-off time.For afixed value of total walk-off time,a smaller dispersion coefficient results in a reduced soliton pulse width.This is because the peak power of an optical soliton pulse is directly proportional to the magnitude of the<<first-order dispersion coefficientand inversely propor-2tional to the square of the soliton pulse width.A reduction in<<peak power,due to lower values of,is partially compen-2sated by the decrease of the soliton pulse width.For lossy Ž.fiber,Eq.6is solved numerically,and the results for a range of␣are also shown in Figure3.With the inclusion of␣,the peak power of a pulse is gradually reduced as the pulse propagates along thefiber loop,and as a result,the phase change due to XPM is also reduced.For␣s0.2dB r km,a narrower pulse width may be used to compensate for the loss of phase due to␣.Ž.Equation8was solved numerically by a combination ofthe function iteration and NRM,and the results for T werei used to calculate the transmission window width.Values of the soliton pulse width and the total walk-off time wereŽ.obtained from Eq.4,and the results for the transmissionwindow width for different values ofare illustrated in2Figure4,showing a threshold level above which the slope increases significantly.The threshold level dependence on the <<can be used as an important parameter to predict the 2width of a soliton switching window.To create a wider switching window,if the total walk-off time is greater than<<the threshold level,then a smaller value ofshould be2used,and if the total walk-off time is smaller than the<<threshold level,then a larger value ofmay be used.By2increasing the width of the switching window,the timing jitter noise can be reduced,but at the cost of increased and interchannel crosstalk by allowing some portion of the adja-cent pulses to be demultiplexed to the output port,which is the subject of further study.Finally,the mathematical model developed is verified by solving the nonlinear Schrodinger equation with the beamŽ.w xpropagation method BPM2,and the results are shown in Table1.An interactivefiber length of3km andfirst-order dispersion parameters of5ps2r km are used for calculation, and the pulse width for loss-free and lossyfiber are obtained Ž.Ž.from Eqs.4and6,respectively with T s10ps.Thet wresults obtained agree very closely,thus verifying the accu-racy of the model developed.However,the deviation of the window width increases as thefiber loss per kilometer increases.4.CONCLUSIONSA mathematical model for NOLM employing a soliton con-trol pulse has been presented,where the problem of pulse deformation during propagation within the loop is overcome. It has been shown that the model can be used to optimize critical parameters,such as walk-off time and pulse width,for maximizing output transmittance with much reduced timing jitter effects.The accuracy of the mathematical model devel-oped is verified by solving the nonlinear Schrodinger equa-tion.ACKNOWLEDGMENTThe work of C.Y.Cheung was supported by an Overseas Research Students Award.REFERENCES1.M.Jinno and T.Matsumoto,Nonlinear Sagnac interferometerŽ.switch and its applications,IEEE J Quantum Electron281992, 875᎐882.2.K.Uchiyama et al.,Effects of control-signal pulse walk-off onBER performance of nonlinear optical loop mirror demultiplexer,Ž.Electron Lett291993,1870᎐1871.3.P.R.Turner,Numerical analysis,Macmillan,New York,1994.4.L.P.Barry et al.,Effect of control pulse deformation on theswitching characteristics of a NOLM,Proc21st Australian Conf Optical Fibre Technol,Dec.1996,pp.197᎐200.5.K.Uchiyama et al.,Signal-to-noise ratio analysis of100Gb r sdemultiplexing using nonlinear optical loop mirror,J Lightwave Ž.Technol151997,194᎐201.6.G.P.Agrawel,Nonlinearfiber optics,Academic Press,New York,1989.ᮊ1999John Wiley&Sons,Inc.CCC0895-2477r99TABLE1Results Using BPM for L=3km and=y5ps2/km2Fiber Loss Mathematical Ž.dB r km Parameter BPM Model 0Transmittance0.9998 1.0000Ž.Window width F s5%ps 6.73 6.75p0.2Transmittance 1.0000 1.0000Ž.Window width F s5%ps 6.66 6.92p0.5Transmittance 1.0000 1.0000Ž.Window width F s5%ps 6.327.17p。
金的等离子共振等综述
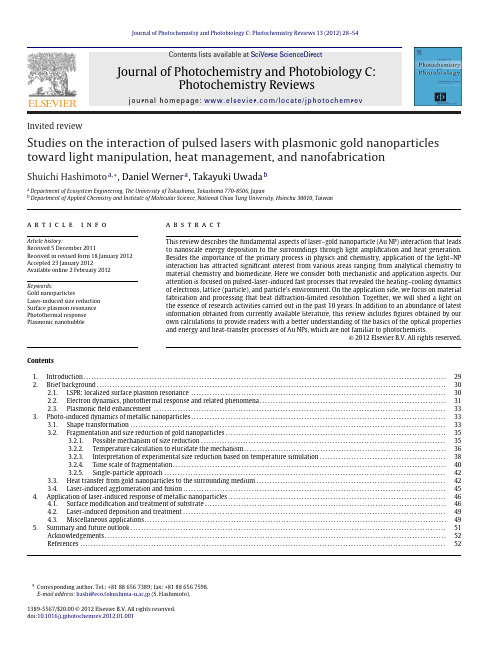
a b s t r a c t
This review describes the fundamental aspects of laser–gold nanoparticle (Au NP) interaction that leads to nanoscale energy deposition to the surroundings through light amplification and heat generation. Besides the importance of the primary process in physics and chemistry, application of the light–NP interaction has attracted significant interest from various areas ranging from analytical chemistry to material chemistry and biomedicine. Here we consider both mechanistic and application aspects. Our attention is focused on pulsed-laser-induced fast processes that revealed the heating–cooling dynamics of electrons, lattice (particle), and particle’s environment. On the application side, we focus on material fabrication and processing that beat diffraction-limited resolution. Together, we will shed a light on the essence of research activities carried out in the past 10 years. In addition to an abundance of latest information obtained from currently available literature, this review includes figures obtained by our own calculations to provide readers with a better understanding of the basics of the optical properties and energy and heat-transfer processes of Au NPs, which are not familiar to photochemists. © 2012 Elsevier B.V. All rights reserved.
CPICPIICPIII测量技术专业知识课件
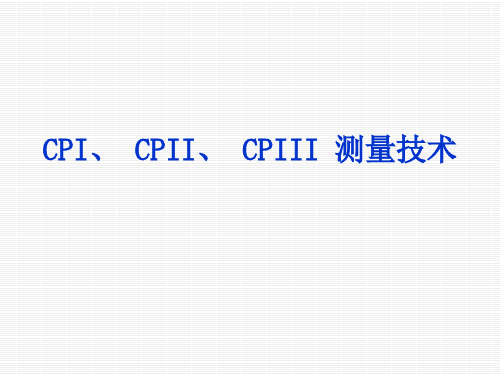
一般要求→三网合一
●勘测控制网是勘测设计单位在勘测设计阶段为满足高速铁路工程勘测设计 和向施工单位进行交桩而建立旳平面、高程控制网,它涉及框架控制网CP0、 基础平面控制网CPⅠ、线路平面控制网CPⅡ和线路水准基点控制网。 ●施工控制网是为高速铁路工程施工提供控制基准旳各级平面高程控制网。 它涉及基础平面控制网CPⅠ、线路平面控制网CPⅡ、线路水准基点控制网, 以及在此基础上加密旳施工平面、高程控制点和为轨道铺设而建立旳轨道控 制网CPⅢ。 ●运营维护控制网是在高速铁路工程竣工后,施工单位交给运营单位,为运 营阶段对高速铁路工程进行变形监测、运营维护旳平面、高程控制网,它涉 及基础平面控制网CPⅠ、线路平面控制网CPⅡ、线路水准基点控制网、轨 道控制网CPⅢ以及轨道维护基标。
CPI、 CPII、 CPIII 测量技术
主要内容
一般要求 基本条件 布网埋标 外业测量 数据处理 CPIII评估
一般要求→规范
《铁路测量技术规则》(分篇),1976年9月出版( 语录版)
↓ 《铁路测量技术规则》(综合)(TBJ101-85) 《既有铁路测量技术规则》(TBJ105-88), 1989年7月1日施行
◆工程独立坐标系
采用任意中央子午线和高程投影面进行投影而建立旳平面直角坐 标系。目旳是控制线路设计高程面上旳投影长度变形值。
◆高程系统采用1985国家高程基准。
一般要求→三网合一
◆三网合一 铁路工程测量旳平面、高程控制网,按施测阶段、施测
目旳及功能可分为勘测控制网、施工控制网、运营维护控制 网。为了确保勘测、施工、运营维护各阶段平面、高程测量 成果旳一致性,提出“三网合一”概念,即:勘测、施工、 运营三个阶段控制网统一。各阶段平面控制测量应以基础平 面控制网(CPⅠ)为基准,高程控制测量应以线路水准基点 控制网为基准。
Bauer Model 920 Peak Limiting Amplifier 说明书
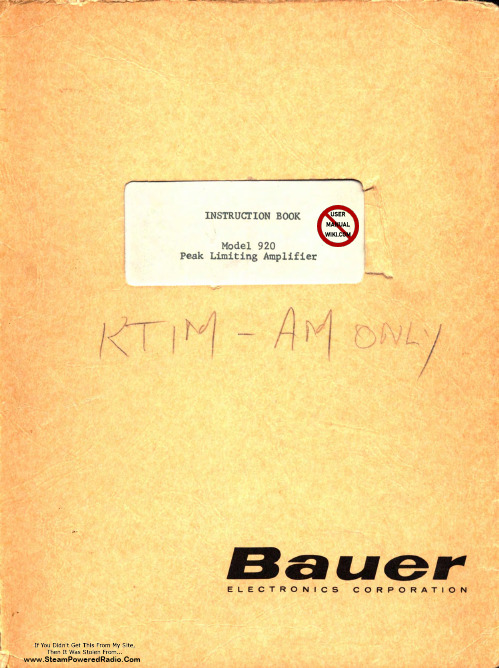
terminal strip also provides for connecting a remote VU meter
I
if desired. Separate adjustments of the attack and release times are provi-
ded on the front panel as well as a limit defeat switch mounted
I
RELEASE TIME: Adjustable from 27 to 527 milliseconds.
I
- TUBE COMPLEMENT: One each, 6BC8, 12AX7, 12BH7 , 6ALS, OB2, GZ34.
DIMENSIONS:
Width: 19 inches.
Height: 3~ inches.
I
ment of its manufacture and assembly for one (1) year against breakage or failure of parts due to imperfection
of workmanship or material, its obligation being limited
gain reduction in db, and input and output attenuators detented
I
in 2 db steps, with vernier adjustments in excess of 2 db for each step.
I
The limiter is provided with a terminal strip on the rear of the unit for interconnecting the amplifier with a system. The
ALTEK模型40A频率源数据表说明书

DATA SHEET MODEL 40A40A Additional Frequency Calibrators: See Data Sheet 942© June 1999 Altek Industries Corp Rochester, NY 14624 USA100891-900 REV:AACCURACY: ±0.0008% (8 parts per million)FREQUENCIES: 5, 6, 10, 12, 15, 20, 30 and 60 Hz times multiplierMULTIPLIER: 0.1, 1, 10, 100 and 1000 times frequency scale OUTPUT: Nominal 15V peak-to-peak square wave (1:3 ratio 20KHz, 2:5 ratio 12KHz)OUTPUT MODES: Switch selected; zero based or zero crossingATTENUATOR: Sliding potentiometer, logarithmic taper OUTPUT IMPEDANCE: 2500 ohms maximumOUTPUT CURRENT DRIVE: 6mA at max output voltage RISE TIME: 1 microsecond nominal into resistive loadOPERATING AMBIENT TEMPERATURE: 14 to 158° F (-10 to +70°C)STORAGE TEMPERATURE: -22 to +185° F (-30 to +85°C)AMBIENT TEMPERATURE EFFECT: ±0.001%/°C BATTERY: 2 x 9-volt AlkalineBATTERY LIFE: Nominal 200 hours of operation. Batteries should be removed when storing the unit for >3 months.BATTERY INDICATION: LED flashes with frequency if batteries OKSIZE: 2-1/8 x 4 x 2-1/4 inches (54x102x55mm)WEIGHT: 7 oz. (0.2 kg)OPTIONAL CARRYING CASE: Zippered with belt loop(unless otherwise indicated, specifications are at 23°C)Specifications are subject to change without noticeSWITCH POSITION MULTIPLIER DESIRED FREQUENCY IN Hz 0.10.50.6 1 1.2 1.5 2 3 6 1 5 6 10 12 15 20 30 60 10 50 60100120150200300600 100500600 1K 1.2K 1.5K 2K 3K 6K 10005K 6K 10K 12K 15K 20K 30K 60K 56101215203060SWITCH POSITION Hz X MULTIPLIERORDERING INFORMATION:MODEL 40A:40A-0560Optional Carrying Case09-3781WARRANTYOur equipment is guaranteed against defective material and workmanship (excluding batteries) for a period of three years from date of shipment. Claims under guarantee can be made by returning the equipment prepaid to our factory. The equip-ment will be replaced, repaired or adjusted at our option.The liability of Altek is restricted to that given under our guarantee. No responsibility is accepted for damage, loss or other expense incurred through sale or use of our equipment. Under no condition shall Altek be liable for any special, incidental or consequential damage.OTHER PRODUCTSAltek designs and manufactures fast, accurate instruments for measurement, generation and simulation of virtually every process control signal. Consult our factory directly or contact your local stocking representative to order precise, low cost Milliamp Calibrators, Voltage Sources, Direct Thermocouple Sources, RTD Simulators and Frequency Sources. Altek also produces calibrators for custom ranges and unique applica-tions. Additional models and ranges are frequently added to the Altek instrument family to meet all of your critical calibration requirements. Altek products are made in the USA.AVAILABLE FROM:。
峰值时间的缩写
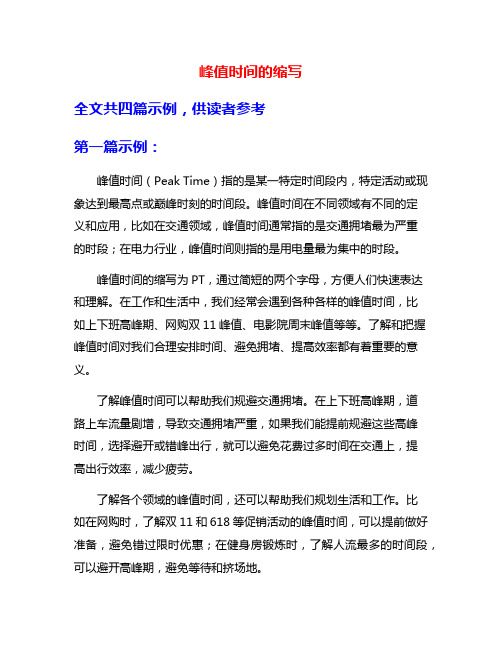
峰值时间的缩写全文共四篇示例,供读者参考第一篇示例:峰值时间(Peak Time)指的是某一特定时间段内,特定活动或现象达到最高点或巅峰时刻的时间段。
峰值时间在不同领域有不同的定义和应用,比如在交通领域,峰值时间通常指的是交通拥堵最为严重的时段;在电力行业,峰值时间则指的是用电量最为集中的时段。
峰值时间的缩写为PT,通过简短的两个字母,方便人们快速表达和理解。
在工作和生活中,我们经常会遇到各种各样的峰值时间,比如上下班高峰期、网购双11峰值、电影院周末峰值等等。
了解和把握峰值时间对我们合理安排时间、避免拥堵、提高效率都有着重要的意义。
了解峰值时间可以帮助我们规避交通拥堵。
在上下班高峰期,道路上车流量剧增,导致交通拥堵严重,如果我们能提前规避这些高峰时间,选择避开或错峰出行,就可以避免花费过多时间在交通上,提高出行效率,减少疲劳。
了解各个领域的峰值时间,还可以帮助我们规划生活和工作。
比如在网购时,了解双11和618等促销活动的峰值时间,可以提前做好准备,避免错过限时优惠;在健身房锻炼时,了解人流最多的时间段,可以避开高峰期,避免等待和挤场地。
对于一些需要抢购或抢单的活动,了解峰值时间更是至关重要。
比如在网约车行业,了解每天的高峰时间,可以提高订单响应速度,增加收入;在电商物流业,了解订单峰值时间,可以合理调配人力资源,保障订单及时送达。
了解和把握峰值时间,可以帮助我们更好地规划生活和工作,提高效率,避免拥堵,减少浪费。
掌握“PT”的含义,对我们的日常生活和工作都有着积极的影响。
希望大家在生活中能够更多地利用峰值时间,让生活更加有序和高效。
【此文2000字,感谢阅读】。
第二篇示例:峰值时间(Peak Time)是指在某一段时间内,某一种活动或现象达到最高水平或最高峰值的时间点。
在生活中,峰值时间可以指购物高峰期、上下班高峰时间、旅游旺季等。
在科技领域,峰值时间则是指网络流量高峰期、服务器负载高峰时段等。
PeakTime参考文件-商业模拟课件-2007PT2
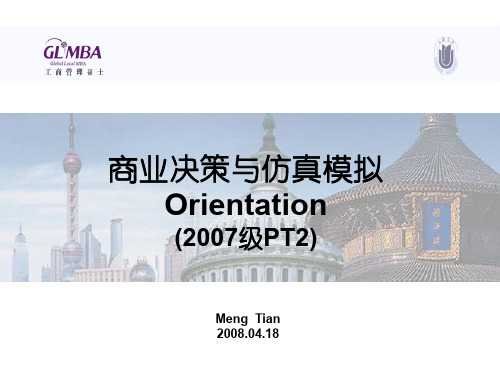
P10
决策制定—市场前景
Ethics, Quality, People, Brand
开始决策制定前,首先要进入“市场前景”模块对市场需求进行分析。
市场需求量的预测
本回合所处的季节情况; 决策制定将根据酒店经 营的季节性划分,分 为夏季和冬季。两个季 节各为六个月的时间。
国内、国际投资市场价 格情况,租赁与投资 国内、国际人力成本情 况,解聘比率 国内、国际税率利率情况 现金银行存款 短期=长期+X 国内、国际市场最新情 况描述 * 也受其他公司决策的影响。
• 投资决策--租 或买/维修 • 成本控制决策 • 人力资源管理
财务决策
• 筹资决策 • 股利分红决 策
• 根据销售、运 营、财务决策 生成预测性财 务报表,供决 策参考
决策列表
• 小组各成员的 决策情况可选 择其中之一作 为小组结果
市场前景
运营决策
财务报表
Our graduates are the global local elite talents you can trust and work with.
Our graduates are the global local elite talents you can trust and work with.
P8
案例介绍—国际市场情况
Ethics, Quality, People, Brand
在国际市场,观光游客是主要的客源,所以季节性需求的变化也很大。国 际市场对酒店的需求瞬息万变,它可以弥补国内市场季节性销售的不足。 在新市场,客房主要通过各种代理商进行销售。即时入住的客人比较少。 由于代理商可以选择的酒店很多,所以广告在营销过程中起了很重要的作 用。关键是要让广告足以吸引代理商。 在国际市场中,工资和成本水平要比国内酒店低;聘用和解聘费用也要比 国内酒店低,因此人力资源管理方面的问题并不像国内那么尖锐。 就整体价格来讲,唯一例外的是,由于地产炒作,国际市场房地产价格达 到了国内市场的同等水平。 由于国际市场中酒店学校提供较好的基础教育,使得其员工工作效率比国 内酒店的员工工作效率高。通过员工培训后,员工的工作效率能够得以提 高。 国际酒店的服务质量没有国内酒店那么高。因为,在这里一些工作上的失 误很容易被原谅。
AEC_Q200-002A
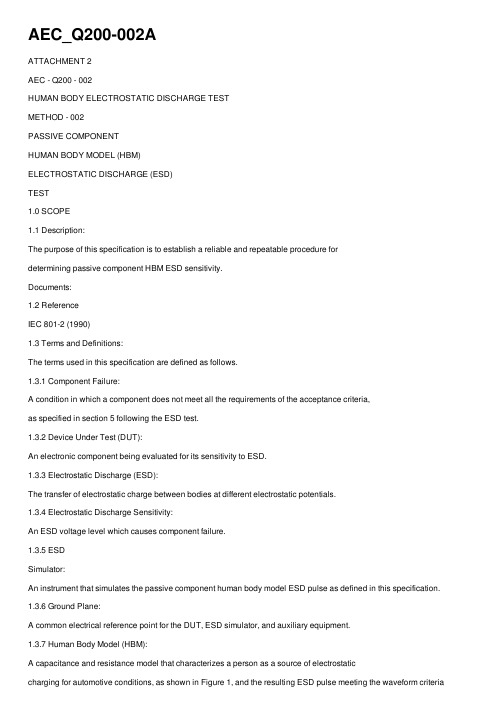
AEC_Q200-002AATTACHMENT 2AEC - Q200 - 002HUMAN BODY ELECTROSTATIC DISCHARGE TESTMETHOD - 002PASSIVE COMPONENTHUMAN BODY MODEL (HBM)ELECTROSTATIC DISCHARGE (ESD)TEST1.0 SCOPE1.1 Description:The purpose of this specification is to establish a reliable and repeatable procedure fordetermining passive component HBM ESD sensitivity.Documents:1.2 ReferenceIEC 801-2 (1990)1.3 Terms and Definitions:The terms used in this specification are defined as follows.1.3.1 Component Failure:A condition in which a component does not meet all the requirements of the acceptance criteria,as specified in section 5 following the ESD test.1.3.2 Device Under Test (DUT):An electronic component being evaluated for its sensitivity to ESD.1.3.3 Electrostatic Discharge (ESD):The transfer of electrostatic charge between bodies at different electrostatic potentials.1.3.4 Electrostatic Discharge Sensitivity:An ESD voltage level which causes component failure.1.3.5 ESDSimulator:An instrument that simulates the passive component human body model ESD pulse as defined in this specification.1.3.6 Ground Plane:A common electrical reference point for the DUT, ESD simulator, and auxiliary equipment.1.3.7 Human Body Model (HBM):A capacitance and resistance model that characterizes a person as a source of electrostaticcharging for automotive conditions, as shown in Figure 1, and the resulting ESD pulse meeting the waveform criteriaspecified in this test method.1.3.8 Maximum Withstanding Voltage:The maximum ESD voltage at which, and below, the component is determined to pass thefailure criteria requirements specified in section 4.1.3.9 PUT:PUT is the pin and/or terminal under test.2.0 EQUIPMENT:2.1Test Apparatus:The apparatus for this test consists of an ESD pulse simulator with an equivalent passivecomponent HBM ESD circuit as shown in Figure 1. The simulator must be capable of supplying pulses that meet the waveform requirements of Table 1 and Figure 3 using the coaxial targetspecified in section 2.2.1.Figure 1: Equivalent Passive Component HBM ESD simulator circuitEquipment:2.2 MeasurementEquipment used to verify conformance of the simulator discharge waveform to the requirements as specified in Table 1 and Figure 3 shall be either an analog oscilloscope with a minimumbandwidth of 1 GHz or a digital oscilloscope with a minimum sampling rate of 2 gigasamples per second and a minimum bandwidth of 1 GHz. Each instrument shall have a 50 ohm inputimpedance. A faster oscilloscope (larger bandwidth and/or higher sampling rate) may berequired to fully characterize the ESD waveform.Target:2.2.1 CoaxialThe coaxial target shall be a current-sensing transducer as specified by IEC 801-2 (1990), orequivalent. The target is used to verify the ESD simulator waveform as defined in sections 2.3,2.3.1, and 2.3.2.2.2.2 Ground Plane:The ground plane is a common electrical reference point with dimensional requirements of a1 mm minimum thickness and a 1 m2 minimum area. The ground plane is connected to earthground by a ground strap as short and as wide as possible; length ≤ 1 m, width ≥ 5 mm, andinductance ≤ 2 µH.2.2.3 Probe:The probe used to verify the ESD simulator charging voltage, as defined in sections 2.3.2 and2.4, shall be an electrometer probe with an input impedance ≥ 100 Gigohm.2.2.4 20 dB Wideband Attenuator:A 20 dB wideband attenuator may be required depending on the vertical sensitivity of theoscilloscope. The attenuator shall be a 50 ohm, 20 dB wideband attenuator with a bandwidth of20 GHz and 2 kW peak pulse power. When using the attenuator, it shall be attached to theoutput of the coaxial target during the ESD simulator qualification and waveform verification as defined in sections 2.3 and 2.4.2.3 ESD Simulator Qualification:ESD simulator calibration and qualification must be performed during initial acceptance testing and whenever the simulator is serviced. A period of six (6) months is the maximum permissible time between full qualification tests. The ESD simulator must meet the waveform parameterrequirements for all voltage levels as defined in Table 1, section 2.3.1, and section 2.3.2. If at any time the waveforms do not meet the requirements of Table 1 and Figure 3, the testing shall be halted until waveforms are in compliance.2.3.1 Simulator Qualification Setup:a. The simulator qualification setup shall be configured according to the equivalentschematic shown in Figure 2. Note that a 20 dB wideband attenuator may be required as shown in Figure 2 depending on the vertical sensitivity of the oscilloscope.b. The coaxial target shall be located on and bonded to the center of the ground plane.The target output shall be connected to the oscilloscope through a 50 ohm double-shielded, high frequency, semi-rigid cable with length ≤ 1 meter. The cable shall not be looped and shall be insulated from the ground plane.c. The horizontal time base and vertical amplifier level of the oscilloscope shall beconfigured in order to view the rise time of the ESD waveform. The horizontal sweepshall be set to single event trigger.d. The ESD simulator high voltage ground shall be directly connected to the ground planeby a grounding strap with length ≤ 1 meter and an inductance ≤ 2 µH. The ESDsimulator shall be set up and operated according to its instruction manual.50 ohms(distributed)2 ohms (distributed)50 ohms Scope InputCoaxial Target20 dB (10X)Attenuator(if applicable)ESD SimulatorGround Plane Ground PlaneFigure 2: Equivalent schematic for Simulator Qualification2.3.2 Simulator Qualification Procedure:a. To calibrate the display voltage of the ESD simulator, adjust the simulator voltage to thedesired level and polarity. With the electrometer in direct contact with the discharge tip(see Figure 1), verify the voltage setting at levels of ± 500 V, ± 1 kV, ± 2 kV, ± 4 kV, ± 8kV, ± 12 kV, ± 16 kV, and ± 25 kV. The electrometer reading shall be within ± 10% forvoltages from 200 V to ≤ 25 kV.b. For Direct Contact Discharge Qualification, discharge to the coaxial target at eachvoltage level and polarity shown in Table 1. Record the rise time and first peak currentvalues and verify the parameters meet the requirements of Table 1. Figure 3 illustratesa typical discharge waveform to a coaxial target. The simulator must meet therequirements of Table 1 and Figure 3 for five (5) consecutive waveforms at all voltagelevels.c. For Air Discharge qualification, the ESD simulator shall be placed a distance of ≥ 15 mmfrom the coaxial target sphere. The ESD simulator, with the air discharge probeattached, shall be held perpendicular (± 15°) to the target. From this position, thesimulator air discharge probe shall be slowly (≤ 5 mm/second) moved towards the targetuntil a single discharge occurs. Only single event discharge waveforms shall beacceptable. Test voltages for the air discharge are ± 12 kV, ± 16 kV, and ± 25 kV.Figure 3 illustrates a typical discharge waveform to a coaxial target. The slow approachmethod specified above minimizes multiple discharges, discharges at lower voltagelevels, and ringing in the measurement equipment.2.4 ESD Simulator Charge Verification:The performance of the simulator can be dramatically degraded by parasitics in the dischargepath. Therefore, to ensure proper simulation and repeatable ESD results, ESD simulator charge verification shall be performed before each daily use. With the electrometer in direct contactwith the discharge tip (see Figure 1), verify the voltage setting at levels of ± 500 V, ± 1 kV, ± 2 kV, ± 4 kV, ± 8 kV, ± 12 kV, ±16 kV, and ± 25 kV. The electrometer reading shall be within ± 10% for voltages from 200 V to ≤ 25 kV. If at any time the simulator charge does not meet the requirements of Table 1, the testing shall be halted until the simulator is in compliance and allESD testing performed since the last passing charge verification shall be considered invalid.Table 1: Direct Contact and Air Discharge ESD waveform parameter requirementsESD Discharge Method IndicatedVoltage(kV)First PeakCurrent, Ip(A)Rise Time,tr(ns)0.5 ± 0.05 1.87 +0.60/-0 0.7 to 1.01.0 ± 0.1 3.75 +1.12/-0 0.7 to 1.0Direct Contact Discharge 2.0 ± 0.5 7.50 +2.25/-0 0.7 to 1.04.0 ± 0.5 15.0 +4.50/-0 0.7 to 1.08.0 ± 0.8 30.0 +9.0/-0 0.7 to 1.0Air Discharge 25.0 ± 2.5 Not Specified Not SpecifiedFigure 3: Typical Direct Contact and Air Discharge PASSIVE COMPONENT HBM ESD discharge waveform to a coaxial targetPROCEDURE:3.0 TESTSize:3.1 SampleEach sample group shall be composed of 15 components, as specified in Table 1 of AEC -Q200. Each sample group shall be stressed at one (1) voltage level using all pin and/or terminal combinations specified in section 3.2. The use of a new sample group for each stress voltage level is recommended. It is permitted to use the same sample group for the next stress level if all components in the sample group meet the acceptance criteria requirements specified insection 5 after exposure to a specified voltage level.3.2 Pin and/or Terminal Combinations:Each pair of pins and/or terminals and all combinations of pin and/or terminal pairs for each component shall be subjected to one (1) pulse at each stress voltage polarity following the ESD levels stated in Figure 4. Any pin and/or terminal not under test shall be in an electrically open (floating) state. A sufficient number of ESD levels must be tested to either: a) demonstrate the component can pass a 25 kV Air Discharge exposure, or b) determine the pass/fail transition region between two (2) consecutive ESD test levels. If an expected failure level cannot be estimated, the test flow diagram of Figure 4 may be used to minimize the amount of testing required.3.3 TestEnvironment:Each component shall be subjected to ESD pulses at 22C ± 5C. For all Air Discharge testing,the relative humidity shall be 30% to 60%.3.4 Measurements:Prior to ESD testing, complete parametric testing (initial electrical verification) shall beperformed on all sample groups and all components in each sample group per applicable userdevice specification at room temperature followed by hot temperature, unless specified otherwise in the user device specification. If using an allowable parametric shift as a failure criterion, adata log of each component shall be made listing the applicable parameter measurement values.The data log will be compared to the parameters measured during final electrical testverification testing to determine the failure criteria of section 4.Setup:3.5 Testa. Ensure that the daily ESD simulator charge verification procedure (section 2.4) has beenperformed before applying discharges to the DUT.b. The ESD simulator high voltage ground shall be directly connected to the ground planeby a grounding strap with a length ≤ 1 meter and an inductance ≤ 2 µH.c. All DUTs are considered sensitive to ESD until proven otherwise and shall be handledaccordingly (reference internal procedures for proper handling of ESD sensitivecomponents).d. The DUT must pass complete parametric testing (initial electrical verification) as definedin section 3.4 prior to any application of ESD stress voltage levels.Procedure:3.6 Detailed3.6.1 Direct Contact Discharge:a. The ESD simulator shall be placed in direct contact with each PUT specified in section3.2.b. Each PUT within a sample group shall be tested at a stress voltage level specified in the test flow diagram of Figure 4 using the Direct Contact discharge probe. Two (2) discharges shall be applied to each PUT within a sample group and at each stress voltage level, one (1) with a positive polarity and one (1) with a negative polarity.c. After each discharge to the PUT, residual charge remaining on the DUT shall be dissipated by briefly connecting a one (1) megohm resistor between the PUT discharge location and/or ground point of the DUT and the ground of the test setup.d. Repeat the procedure for all components within the sample group.e. Using the next sample group, select another direct contact stress voltage level and repeat the above procedure until all sample groups have been tested at a specified voltage level. It is permitted to use the same sample group for the next stress voltage level if all components in the sample group meet the acceptance criteria requirements specified in section 5 after exposure to a specified voltage level.f. Submit the components for complete parametric testing (final electrical verification) per the user device specification at room temperature followed by hot temperature, unless specified otherwise in the user device specification, and determine whether the components meet the acceptance criteria requirements specified in section 5. It is permitted to perform the parametric testing (final electrical verification) per user device specification after all sample groups have been tested.Discharge:3.6.2 Aira. Using a new sample group, each PUT specified in section 3.2 shall be tested at an air discharge stress voltage level specified in the test flow diagram of Figure 4 using the Air Discharge probe. It is permitted to use the same sample group for the next stressvoltage level if all components in the sample group meet the acceptance criteria requirements specified in section 5 after exposure to a specified voltage level.b. The ESD simulator shall be placed ≥ 15 mm away from the PUT. The simulator, with the Air Discharge probe, shall be held perpendicular to the PUT discharge location. The probe shall be slowly moved towards the PUT (e.g., ≤ 5 mm/second) until a single discharge is obtained.c. If no discharge occurs, continue moving the probe towards the DUT until the simulator discharge probe contacts the PUT discharge location. If the simulator makes contactwith the PUT discharge location and discharge occurs, discontinue testing at the stress voltage level and location. A test board with closely spaced lead wires or metal runnersmay prevent discharging to an intended PUT and result in an arcing phenomenon.When this situation occurs, multiple test boards with a reduced number of lead wires or metal runners shall be used.d. Two (2) discharges shall be applied to each PUT within a sample group at the air discharge stress voltage level, one (1) with a positive polarity and one (1) with a negative polarity.e. After each discharge to the PUT, residual charge remaining on the DUT shall be dissipated by briefly connecting a one (1) megohm resistor between the PUT discharge location and/or ground point of the DUT and the ground of the test setup.f. Repeat the procedure for all components within the sample group.g. Using the next sample group, select another air discharge stress voltage level and repeat the above procedure until all sample groups have been tested at a specificvoltage level. It is permitted to use the same sample group for the next stress voltagelevel if all components in the sample group meet the acceptance criteria requirements specified in section 5 after exposure to a specified voltage level.h. Submit the components for complete parametric testing (final electrical verification) per the user device specification at room temperature followed by hot temperature, unless specified otherwise in the user device specification, and determine whether the components meet the acceptance criteria requirements specified in section 5. It is permitted to perform the parametric testing (final electrical verification) per user device specification after all sample groups have been tested.4.0 FAILURECRITERIAA component will be defined as a failure if, after exposure to ESD pulses, the component fails any of the following criteria:1. The component exceeds the allowable shift value. Specific parameters and allowable shift values shall be defined in the applicable user device specification. During initial parametric testing, a data log shall be made for each component listing the applicable parameter measurement values. The data log will be compared to the parameters measured during final parametric testing to determine the shift value. Components exceeding the allowable shift value will be defined as a failure.2. The component no longer meets the user device specification requirements. Complete parametric testing (initial and final electrical verification) shall be performed perapplicable user device specification.CRITERIA:5.0 ACCEPTANCEA component passes a voltage level if all components stressed at that voltage level pass. All the samples used must meet the measurement requirements specified in section 3 and the failure criteria requirements specified in section 4. Using the classification levels specified in Table 2, classify the components according to the highest ESD voltage level survived during ESD testing. The supplier shall define the ESD withstanding voltage for each component. Table 2: Passive Component HBM ESD classification levels(DC = Direct Contact Discharge, AD = Air Discharge)Component Classification Maximum Withstand Voltage1A < 500 V (DC)1B 500 V (DC) to < 1000 V (DC)1C 1000 V (DC) to < 2000 V (DC)2 2000 V (DC) to < 4000 V (DC)3 4000 V (DC) to < 6000 V (DC)4 6000 V (DC) to < 8000 V (DC)5A 8000 V (DC) to < 12,000 V (AD)5B 12,000 V (AD) to < 16,000 V (AD)5C 16,000 V (AD) to < 25,000 V (AD)6 ≥ 25,000 V (AD)FAIL PASSDirect Contact6 kVPASSFAIL FAIL PASS FAIL PASSFAIL PASS < 500 V DC Direct Contact500 VDirect Contact1 kVDirect Contact4 kVDirect Contact2 kV500 V DC 1 kV DC 2 kV DC 4 kV DC FAIL PASS> 25 kV AD Air Discharge25 kV16 kVDirect Contact8 kV12 kV12 kV AD8 kV DC 6 kV DC 16 kV ADAir DischargeAir DischargeFAIL PASSFAIL PASS FAIL PASSNote 1: Classify the components according to the highest ESD voltage level survived during ESD testing.Figure 4: Passive Component HBM ESD test flow diagram(DC = Direct Contact Discharge, AD = Air Discharge)Revision HistoryRev # Date of change Brief summary listing affected paragraphsApril 30, 1996 Initial Release.A March 15, 2000 Removed CDF designation through document.Removed Chrysler, Delco, and Ford logo from each heading.Add Component Technical Committee to each heading.。
- 1、下载文档前请自行甄别文档内容的完整性,平台不提供额外的编辑、内容补充、找答案等附加服务。
- 2、"仅部分预览"的文档,不可在线预览部分如存在完整性等问题,可反馈申请退款(可完整预览的文档不适用该条件!)。
- 3、如文档侵犯您的权益,请联系客服反馈,我们会尽快为您处理(人工客服工作时间:9:00-18:30)。
预售
在以后两个回合的预先销售中,你要决定有多少客房天数将供应给旅行社和代 理处。它会直接影响到你的预售客房价格。
Our graduates are the global local elite talents you can trust and work with.
P13
决策制定—运营决策
国内市场中,酒店已经拥有 了100年的租赁合同。酒 店拥有目前建筑的使用权。 所以酒店要自行负担其新的 投资建设费用。 对客房的所有投资都需要一 个回合的时间才能完成。 装修可以提高酒店的整体质 量。较好的设施条件被视为 较好的酒店质量。 如果你决定拓展国际市场, 你需要决定选择在土地租赁 合约中增加酒店大楼租赁, 还是自己负担新酒店大楼的 建设。你必须选择其一来开 启国际市场运营。 在租赁合同中增加租用酒店 大楼,意味着你将支付酒店 大楼的租金
Ethics, Quality, People, Brand
开始决策制定前,首先要进入“市场前景”模块对市场需求进行分析。
市场需求量的预测
本回合所处的季节情况; 决策制定将根据酒店经 营的季节性划分,分 为夏季和冬季。两个季 节各为六个月的时间。
国内、国际投资市场价 格情况,租赁与投资 国内、国际人力成本情 况,解聘比率 国内、国际税率利率情况 现金银行存款 短期=长期+X 国内、国际市场最新情 况描述 * 也受其他公司决策的影响。
Our graduates are the global local elite talents you can trust and work with.
P3
什么是Cesim OnService?
Ethics, Quality, People, Brand
Our graduates are the global local elite talents you can trust and work with.
商业决策与仿真模拟 Orientation
(2007级PT2)
Meng Tian 2008.04.18
课程目标
Ethics, Quality, People, Brand
The aim of the business simulationⅡ is that the student teams will manage the virtual Service company (Hotel) through technological evolution in the fast-paced 21st century business environment. The service industry is very different from manufacture industry which was operated by students in Business SimulationⅠ.They will have the opportunity to manage local Market and develop fresh international Market. Students will manage the company’s development activities, global production, Sales and Market, Human Resources Management and Finance operations as they develop and execute a solid strategy for their virtual company. Please pay attention: we establish 7 rounds in this simulation.
系统时间和决策小组 所处时区的时间
P6
案例介绍—酒店业务情况
Ethics, Quality, People, Brand
决策制定将根据酒店经营的季节性分为夏季和冬季。每个季节为六个月的时 间。酒店目前有50间客房,计划供休闲旅客入住。 商务旅客也时常入住酒店,尤其是在冬季。由于休闲旅客主要在夏季入住酒 店,而商务旅客在任何季节都会入住酒店,所以在淡季的时候商务旅客受到 了更多的欢迎。 酒店的利润主要来源于旺季的销售,淡季的经营方针是避免亏损,尽量做到 收支平衡。 酒店将通过各种销售渠道将预售部分房间。管理层要事先制定出一年(二个 季节)和半年(一个季节)预售的客房数量。 代理商会在成本价(他们付给酒店的价钱)和销售价(他们给最终用户的价 格)之间获利,所以他们对数量较为敏感。根据你提供的数量,他们会决定 预售房间的价格.在市场未饱和的情况下,预售数量由你决定。 在每个回合中,你要决定即时入住客人的房价,在与竞争者的价格比较后, 得到客房需求量。除了价格之外,市场需求量也受酒店运营水平影响。
P4
案例介绍—背景知识
Ethics, Quality, People, Brand
Our graduates are the global local elite talents you can trust and work with.
P5
什么是Cesim OnService?
Ethics, Quality, People, Brand
Our graduates are the global local elite talents you can trust and work with.
P2
课程目标
Ethics, Quality, People, Brand
Students are the management team of Hotel Company and compete with other teams to gain market share and create value for the shareholders. The objective of the game is to achieve the highest financial performance through a sound business strategy, timely decisions, and accurate implementation. Strategic approach to decision-making, careful analysis, good Marketing and successful product positioning are the main keys to success.
销售
-
Ethics, Quality, People, Brand
你可以提前两个回合把客房销售给旅行社和网上预定代理处,预先销售下一回 合的客房以(+1)表示,预先销售再下一个回合的客房以(+2)表示。 你需要为本回合那些未经预订的客房定价。 一 般来说,预订客房的客人要比那些即来即住的客人对价格更为敏感。
• 投资决策--租 或买/维修 • 成本控制决策 • 人力资源管理
财务决策
• 筹资决策 • 股利分红决 策
• 根据销售、运 营、财务决策 生成预测性财 务报表,供决 策参考
决策列表
• 小组各成员的 决策情况可选 择其中之一作 为小组结果
市场前景
运营决策
财务报表
Our graduates are the global local elite talents you can trust and work with.
Ethics, Quality, People, Brand
Our graduates are the global local elite talents you can trust and work with.
P14
决策制定—运营决策
上一回合各小组经营的房价 和酒店质量离散图。可作为 本回合,酒店经营定位参考。 员工数和销售客房天数比较 图。本回合根据客房销售情 况,你应该雇佣多少员工。 酒店的灵活性并不要求所有 的员工需要完成所有的工作。 也与员工压力表有关。 成本控制以降低成本,提 高流程效率为目的。它会对 运作和管理成本产生影响。 第一个成本控制项目会影响 直接服务成本,第二个会影 响固定管理成本。 你可以对成本控制项目重新 命名。 损益表中的管理成本是外包 给其他公司的。
Our graduates are the global local elite talents you can trust and work with.
P11
决策制定—销售决策
当季销售价格
Ethics, Quality, People, Brand
国内、国际市 场的选择 由于受竞争的 影响,实际销 售的天数是不 定的。请尽量 在蓝色格中填 入较为准确的 销售预测。 广告不仅对本 回合产生影响, 同时还有长期 效应。 广告会增加本 回合和今后两 个回合的销售。
Our graduates are the global local elite talents you can trust and work with.
P8
案例介绍—国际市场情况
Ethics, Quality, People, Brand
在国际市场,观光游客是主要的客源,所以季节性需求的变化也很大。国 际市场对酒店的需求瞬息万变,它可以弥补国内市场季节性销售的不足。 在新市场,客房主要通过各种代理商进行销售。即时入住的客人比较少。 由于代理商可以选择的酒店很多,所以广告在营销过程中起了很重要的作 用。关键是要让广告足以吸引代理商。 在国际市场中,工资和成本水平要比国内酒店低;聘用和解聘费用也要比 国内酒店低,因此人力资源管理方面的问题并不像国内那么尖锐。 就整体价格来讲,唯一例外的是,由于地产炒作,国际市场房地产价格达 到了国内市场的同等水平。 由于国际市场中酒店学校提供较好的基础教育,使得其员工工作效率比国 内酒店的员工工作效率高。通过员工培训后,员工的工作效率能够得以提 高。 国际酒店的服务质量没有国内酒店那么高。因为,在这里一些工作上的失 误很容易被原谅。