Cui_nl101884h_Ultrathin Topological Insulator Bi2Se3 by AFM
Ti-Cu-Ni三元大块非晶合金的制备与力学性能

Txl 500 550 600 650 700 750 800 850
Temperature/K
图 2 Ti50Cu50-xNix(x=5, 8, 11)合金的DSC曲线 Fig.2 DSC traces of Ti50Cu50-xNix glassy alloys (x=5, 8, 11)
综上,目前还没有简单的两元或三元的 Ti 基大块 非晶出现,只制得复杂的多组元大块非晶且其中大都 含有能产生有毒蒸气的 Be 元素。简单的两元或三元 大块非晶合金体系的制备对非晶形成能力的研究有重 大意义。本实验将研究三元 Ti-Cu-Ni 合金系的非晶形 成能力、热稳定性、力学性能和断裂行为,探讨该类 合金制备大块非晶合金的可能性。
第 37 卷 2008 年
第7期 7月
稀有金属材料与工程
RARE METAL MATERIALS AND ENGINEERING
Vol.37, No.7 July 2008
Ti-Cu-Ni 三元大块非晶合金的制备与力学性能
孟力凯,司 颐,索忠源,武晓峰
(辽宁工业大学,辽宁 锦州 121001)
摘 要:采用铜模喷铸法成功制备出成本较低、直径为 2 mm 的 Ti 基 Ti50Cu42Ni8 大块非晶合金。利用 XRD、DSC 及 SEM 等手段研究了 Ti50Cu50-xNix(x=5, 8, 11)合金的非晶形成能力、力学性能与变形行为。结果表明:Ti50Cu42Ni8 大块非 晶合金具有明显且较高的玻璃转变温度 Tg(659 K)、晶化初始温度 Tx(716 K)、宽的过冷液相区 ∆Tx=Tx-Tg(57 K)、高的 约化玻璃转变温度 Trg=Tg/Tm(0.565)和压缩断裂强度(2008 MPa)。
Thermal stability of ultrafine-grained copper during high speed micro-extrusion
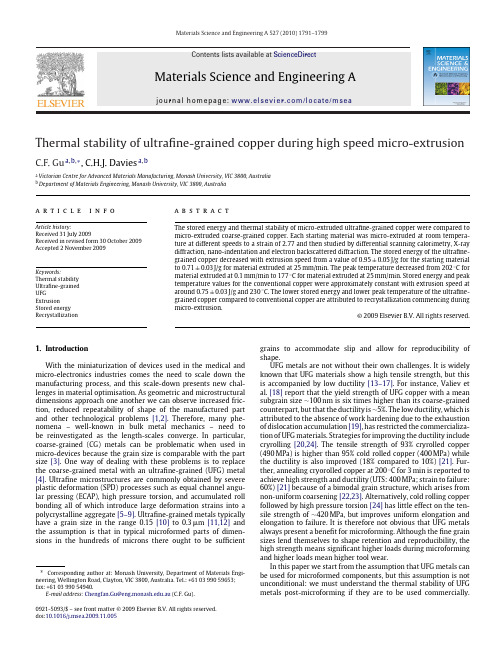
Materials Science and Engineering A 527 (2010) 1791–1799Contents lists available at ScienceDirectMaterials Science and EngineeringAj o u r n a l h o m e p a g e :w w w.e l s e v i e r.c o m /l o c a t e /m s eaThermal stability of ultrafine-grained copper during high speed micro-extrusionC.F.Gu a ,b ,∗,C.H.J.Davies a ,ba Victorian Centre for Advanced Materials Manufacturing,Monash University,VIC 3800,Australia bDepartment of Materials Engineering,Monash University,VIC 3800,Australiaa r t i c l e i n f o Article history:Received 31July 2009Received in revised form 30October 2009Accepted 2November 2009Keywords:Thermal stability Ultrafine-grained UFGExtrusionStored energy Recrystallizationa b s t r a c tThe stored energy and thermal stability of micro-extruded ultrafine-grained copper were compared to micro-extruded coarse-grained copper.Each starting material was micro-extruded at room tempera-ture at different speeds to a strain of 2.77and then studied by differential scanning calorimetry,X-ray diffraction,nano-indentation and electron backscattered diffraction.The stored energy of the ultrafine-grained copper decreased with extrusion speed from a value of 0.95±0.05J/g for the starting material to 0.71±0.03J/g for material extruded at 25mm/min.The peak temperature decreased from 202◦C for material extruded at 0.1mm/min to 177◦C for material extruded at 25mm/min.Stored energy and peak temperature values for the conventional copper were approximately constant with extrusion speed at around 0.75±0.03J/g and 230◦C.The lower stored energy and lower peak temperature of the ultrafine-grained copper compared to conventional copper are attributed to recrystallization commencing during micro-extrusion.© 2009 Elsevier B.V. All rights reserved.1.IntroductionWith the miniaturization of devices used in the medical and micro-electronics industries comes the need to scale down the manufacturing process,and this scale-down presents new chal-lenges in material optimisation.As geometric and microstructural dimensions approach one another we can observe increased fric-tion,reduced repeatability of shape of the manufactured part and other technological problems [1,2].Therefore,many phe-nomena –well-known in bulk metal mechanics –need to be reinvestigated as the length-scales converge.In particular,coarse-grained (CG)metals can be problematic when used in micro-devices because the grain size is comparable with the part size [3].One way of dealing with these problems is to replace the coarse-grained metal with an ultrafine-grained (UFG)metal [4].Ultrafine microstructures are commonly obtained by severe plastic deformation (SPD)processes such as equal channel angu-lar pressing (ECAP),high pressure torsion,and accumulated roll bonding all of which introduce large deformation strains into a polycrystalline aggregate [5–9].Ultrafine-grained metals typically have a grain size in the range 0.15[10]to 0.3m [11,12]and the assumption is that in typical microformed parts of dimen-sions in the hundreds of microns there ought to be sufficient∗Corresponding author at:Monash University,Department of Materials Engi-neering,Wellington Road,Clayton,VIC 3800,Australia.Tel.:+610399059653;fax:+610399054940.E-mail address:Chengfan.Gu@.au (C.F.Gu).grains to accommodate slip and allow for reproducibility of shape.UFG metals are not without their own challenges.It is widely known that UFG materials show a high tensile strength,but this is accompanied by low ductility [13–17].For instance,Valiev et al.[18]report that the yield strength of UFG copper with a mean subgrain size ∼100nm is six times higher than its coarse-grained counterpart,but that the ductility is ∼5%.The low ductility,which is attributed to the absence of work hardening due to the exhaustion of dislocation accumulation [19],has restricted the commercializa-tion of UFG materials.Strategies for improving the ductility include cryrolling [20,24].The tensile strength of 93%cryrolled copper (490MPa)is higher than 95%cold rolled copper (400MPa)while the ductility is also improved (18%compared to 10%)[21].Fur-ther,annealing cryorolled copper at 200◦C for 3min is reported to achieve high strength and ductility (UTS:400MPa;strain to failure:60%)[21]because of a bimodal grain structure,which arises from non-uniform coarsening [22,23].Alternatively,cold rolling copper followed by high pressure torsion [24]has little effect on the ten-sile strength of ∼420MPa,but improves uniform elongation and elongation to failure.It is therefore not obvious that UFG metals always present a benefit for microforming.Although the fine grain sizes lend themselves to shape retention and reproducibility,the high strength means significant higher loads during microforming and higher loads mean higher tool wear.In this paper we start from the assumption that UFG metals can be used for microformed components,but this assumption is not unconditional:we must understand the thermal stability of UFG metals post-microforming if they are to be used commercially.0921-5093/$–see front matter © 2009 Elsevier B.V. All rights reserved.doi:10.1016/j.msea.2009.11.0051792 C.F.Gu,C.H.J.Davies/Materials Science and Engineering A527 (2010) 1791–1799We compare the annealing response and mechanical behavior of ultrafine-grained and coarse-grained(CG)oxygen-free high con-ductivity(OFHC)copper that was micro-extruded at different speeds.We use this comparison to draw inferences about the microstructural evolution during the micro-extrusion of UFG cop-per.In particular micro-extrusion of UFG copper resulted in a reduc-tion of stored energy concurrent with an unexpected acceleration of recrystallization kinetics at high extrusion speeds.This is attributed to recrystallization commencing during micro-extrusion.2.ExperimentalOFHC copper was heat treated at650◦C for2h to a grain size of∼24m and billets of the annealed copper were machined to 20mm square cross-section and processed by ECAP for8passes, route B C(+90◦rotation about the billet long axis for each successive pass)with a die angle of90◦,and back pressure of25MPa.Previous work[25]demonstrates that the resulting UFG material possesses a band-like lamellar structure with the long axis parallel to the macro-shear direction imposed by the ECAP process.The average subgrain size is∼0.23m,within the range of other reported val-ues of about0.3m[11,12]and0.15m[10]obtained by TEM. The resulting ultrafine-grained material was extruded from billets of2mm diameter to extrudate of0.5mm diameter(homogeneous strain,2.77)through a conical die of die angle60◦at four differ-ent speeds of0.1,1,10and25mm/min using a micro-extrusion device in an Instron5500R.Samples were stored in a conventional freezer after extrusion until further testing.Billets of the annealed coarse-grained copper were extruded under the same conditions for comparison.The stored energy and recrystallization temperatures of the UFG and CG material were determined by differential scanning calorimetry using a PerkinElmer DSC7at a constant heating rate of 10K/min in an N2atmosphere,with temperature ranged from50 to300◦C,and at least two samples in the weight range40–85mg being measured.The calorimeter was calibrated using standard Zinc and Indium samples prior to measurement.Following this,a fully recrystallized copper sample was scanned at the same heating rate to provide a baseline reference for each DSC run so that the heat evolution detected is only from the restoration processes taking place in each sample.The stored energy of the deformed materials was calculated using the accompanying commercial software.The hardness of the UFG and CG copper was measured at a constant loading rate of100N/s using a nano-indentation device (TriboLab,Hysitron Incorporated,USA)equipped with a diamond Berkovich tip;at least20indents were performed for each sample. The unloading curves were shifted to the end of the applied max-imum loads of5000N andfitted with a power law between20% and80%of the maximum load.Dislocation density was calculated from peak broadening (microstrain)analysis of X-ray diffraction profile lines by the Mul-tiple Whole Profile(MWP)fitting procedure[26].A high resolution Phillips1140diffractometer operated at40kV and25mA using CuK␣1radiation( =0.1540nm)was used to gather the X-ray peak data;the instrumental broadening was determined to be0.002◦and therefore instrumental correction was not performed.The dis-location density measured in UFG and CG materials can be related to =2/ (b×d)2[27],where b is the Burgers vector(=0.256nm).The microstructure of the materials was characterized by elec-tron backscattered diffraction(EBSD).Specimens were cut from the centre of the extrudate and mechanically polished to4000-grit SiC paper,and then electro-polished in an electrolyte of25% orthophosphoric acid,25%ethanol and50%distilled water at10V, 20◦C with a current of∼150mA.The EBSD measurements were performed using a JEOL7001F FEG SEMfitted with a HKL detector and at least3maps(∼500grains)were measured per extrusion condition.The raw EBSD data was processed by orientation aver-aging using the VMAP software[28]to reduce orientation noise inherent in the data.After noise reduction,the angular resolution was improved to1◦[29].Further analysis of the data was carried out using HKL software(Channel5).In determining the mean boundary intercept(dÂ≥1◦)and misorientation(Â),boundaries with misori-entation lower than1◦were excluded.In contrast with deformation dominated by dislocation glide in grain interiors,boundary related deformation may result in distinct boundary properties.Conse-quently the rotation axes of the boundaries were plotted in sample coordinates and crystal coordinates at each condition examined.3.ResultsThe DSC curves(Fig.1)of the UFG copper show for the start-ing material an exothermic peak which broadens with increasing extrusion speed.The peak temperature(T p)for the starting mate-rial occurred at about163◦C,increased to202◦C after extrusion at0.1mm/min,and decreased with further increasing extrusion speed to about177◦C after extrusion at25mm/min(Fig.2a).Sim-ilar to the trend in peak temperature,the stored energy decreased with increasing extrusion speed from approximately0.95±0.05J/g after8pass ECAP(Fig.2b),which is close to the values for copper processed by ECAP to similar strains[30,10],to0.71±0.03J/g after extrusion at25mm/min.This trend is commonly observed by oth-ers[31,32].The DSC peak temperature of the CG material showed no significant change with increasing extrusion speed(Fig.2a).The stored energy remained roughly constant with extrusion speed at 0.75±0.05J/g,which is∼20%lower than the value of the initial UFG sample(Fig.2b).The hardness of the UFG copper prior to extrusion is close to reported values for ultrafine-grained copper[33]at1.7±0.01GPa. The hardness increased significantly after extrusion at the lower speeds of0.1and1mm/min.After extrusion at25mm/min a substantial decrease in hardness was observed(Fig.3).The hard-ness of the CG material remains approximately constant,declining only slightly after extrusion at25mm/min.The dislocation density (Fig.4)of the UFG copper(19.56±0.38×1014m−2)is similar to reported values[34,35]and followed the same trend as the hard-ness data:an obvious decrease in dislocation densities was found in UFG copper and only slight decrease in CG copper(13.8±0.5to 10.2±1.1×1014m−2).After extrusion the microstructure of the coarse-grained copper elongates in the extrusion direction as expected(Fig.5).The sub-grain size(d ECD)of the CG copper after extrusion lies in the range from∼0.3to0.4m(Fig.10b)and with the low angle boundary fraction is about∼90%.However,∼35%is high angle boundary in UFG/ECAPed copper,close to reported value of∼30%[10–12],Fig.1.DSC curves of UFG and CG copper,and UFG and CG copper extruded at0.1, 1,10,and25mm/min.Samples were scanned at linear heating rate of10K/min. (Bottom-most scans are the unextruded starting materials.)C.F.Gu,C.H.J.Davies/Materials Science and Engineering A527 (2010) 1791–17991793Fig.2.(a)Peak temperatures from DSC measurement for UFG and CG copper;(b) stored energy from DSC measurement for UFG and CG copper.Values for the unex-truded starting material in both cases are shown as an extrusion speed of zero for comparison.and approximately constant at different speeds extrusion.Sub-grain sizes(∼0.25–0.30m)are approximately constant when UFG copper has been extruded at the speeds of0.1,1and10mm/min (Fig.11b),but the elongated grains have clearly recrystallized at25mm/min resulting in an average subgrain size of0.53m (Fig.6e).Turning to the inverse polefigures(IPF)we see two sharpfibre textures developed during extrusion of the CG material,a 111 and 100 fibre(Fig.7b–e).Similar results are reported for the extrusion of fcc metals[36–39],where thefibre developed depends on the stacking fault energy.Materials with a high SFE result in a strong 111 fiber whereas materials with low SFE depicts a strong 100 fibre;copper is found to have a balance of the twofibre com-ponents[40].The samefibre components are found for theUFGFig.3.Nano-indentation hardness variation for extruded UFG and CG copper.Values for the unextruded starting material in both cases are shown as an extrusion speed of zero forcomparison.Fig.4.Dislocation density calculated from XRD measurement for extruded UFG and CG copper.Values for the unextruded starting material in both cases are shown as an extrusion speed of zero for comparison.material extruded at each of0.1,1and10mm/min,but the inten-sity is higher than the CG(Fig.8a–d).After extrusion of the UFG material at25mm/min the intensity decreased almost to that of a random texture(Fig.8e).4.DiscussionThe dislocation densities determined by X-ray measure-ment are close to the dislocation densities obtained by others [30,34,35,41–44]for copper of similar or unknown purity processed by severe plastic deformation(Fig.9).From these data we can calculate the stored energy and compare with the stored energy from all boundaries computed from the EBSD data.There will be some difference due to dislocations between boundaries,but this contribution will be small,as the density between boundaries has previously been shown to be an order of magnitude lower than the values calculated from XRD[29].Eqs.(1)and(2)are used to determine the stored energy from the dislocation density(XRD) and boundary contribution(EBSD),respectively.E d=12Gb2(1) E b=2¯d0Â≥1(2)Applying Eqs.(1)and(2)wefind that the stored energies are consistent within experimental error(Table1).The remaining discrepancy with the DSC stored energy is a contribution from vacancies[25,45,46].The mechanical properties of the CG material and their proxy (dislocation density)are consistent with the annealing response during DSC:the hardness and dislocation densities barely change with increasing extrusion speed and this trend is mirrored in the annealing measures(stored energy and peak temperature).How-ever,in the case of the UFG material,the trend in DSC response with increasing extrusion speed is unusual.Whereas the stored energy declines in concert with the hardness and dislocation density,the peak temperature unexpectedly also declines.We hypothesize that deformation heating during micro-extrusion of the UFG copper is sufficient to initiate restoration mechanisms that then serve to reduce the stored energy and peak temperature during subsequent post-extrusion DSC.To justify this hypothesis we must explore the factors controlling the kinetics of recrystallization and whether the temperature rise during extrusion is sufficient to induce restoration processes.The kinetics of recrystallization is governed by the relation:X∝•N•G(3)1794 C.F.Gu,C.H.J.Davies /Materials Science and Engineering A527 (2010) 1791–1799Fig.5.Representation microstructure of CG copper extruded at different speeds:(a)CG as-received,(b)0.1mm/min,(c)1mm/min,(d)10mm/min and (e)25mm/min (low angle boundaries (1◦≤Â≤15◦)and high angle boundaries (Â>15◦)are depicted by thin gray line and thick black lines,respectively).Subgrains are distinguished by random colors.ED is the extrusion direction and RD radial direction.where X is volume fraction of recrystallization,•N is the rate of nucleation,and •G is interface migration rate:•G ∝MP(4)and P is the driving force,and M the mobility of the recrystallization front [47].In this exposition we first look at the factors that control recrys-tallization during the continuous heating DSC experiment and then seek to explain the effect of prior restoration mechanisms on theseTable 1Stored energy calculation from EBSD and XRD measurement of UFG and CG copper extruded at different speeds.UFG CGInitial0.1mm/min 1mm/min 10mm/min 25mm/min as-received 1mm/min 10mm/min 25mm/min EBSDd ECD m 0.26±0.020.33±0.010.33±0.020.26±0.030.53±0.010.38±0.010.33±0.030.31±0.040.4±0.05 (J/m 2)0.50.520.540.550.560.40.410.420.43E b (J/g)0.430.350.370.470.240.230.280.300.24XRDE d (J/g)0.340.400.400.320.280.250.230.190.18C.F.Gu,C.H.J.Davies/Materials Science and Engineering A527 (2010) 1791–17991795Fig.6.Representation microstructure of UFG copper extruded at different speeds:(a)Initial UFG,(b)0.1mm/min,(c)1mm/min,(d)10mm/min and(e)25mm/min.Note that the scale bar in these images is2m,compare to the scale bar of10m length in Fig.5b–e.1796 C.F.Gu,C.H.J.Davies /Materials Science and Engineering A527 (2010) 1791–1799Fig.7.IPFs of CG as-received copper and CG copper extruded at different speeds:(a)CG as-received,(b)CG extruded at 0.1mm/min,(c)at 1mm/min,(d)at 10mm/min,and (e)at 25mm/min.factors.The DSC response is connected to the recrystallization kinetics by the peak temperature,T p ,which is the temperature at which 50%recrystallization has occurred;during continuous heat-ing accelerated recrystallization leads to a lower peak temperature [48].Thus in order to observe a decreased peak temperature either •N must increase and/or •G must increase (Eq.(3)).We can eliminate an increase in the interface migration rate by looking at the factors that control •G (Eq.(4)).In order to induce an increase in the interface migration rate either the stored energy,P ,or the mobility,M ,must increase.We have shown that the energy stored during extrusion and released by recrystallization during continuous heating DSC decreases (Fig.1)for UFG copper,and thus would expect on this basis that the interface migration rate should also decrease,leading to a higher T p .Turning to mobility,we assume that mobility is an increasing function of misorientation for low angle boundaries and constant for high angle boundaries [47].The average misorientation for low angle boundaries remains approxi-mately constant (Fig.11a)and therefore for a common DSC heating rate the mobility is likely to increase at the same rate for each extru-sion condition.Thus we expect the growth rate to decrease,which would also lead to a higher T p .The remaining possibility is that the nucleation term must benefit from prior restoration mechanisms and our experimental evidence,bolstered by the literature,supports this conclusion.IfFig.8.IPFs of initial UFG copper and UFG copper extruded at different speeds:(a)initial UFG,(b)UFG extruded at 0.1mm/min,(c)at 1mm/min,(d)at 10mm/min,and (e)at 25mm/min.C.F.Gu,C.H.J.Davies /Materials Science and Engineering A 527 (2010) 1791–17991797parison of the deformation induced dislocation densities in severe plastic deformed copper by X-ray measurement [30,34,35,41],DSC measurement [42]and TEM [43,44].grains were to nucleate during micro-extrusion we would expect to observe several differences after extrusion between the high speed and low speed extrusions:(i)an increase in the mean grain size;(ii)a decrease in the stored energy because dislocations have been eliminated;(iii)a decrease in the dislocation density;(iv)an increase in the average misorientation of low angle boundaries.The first three of these four predictions are found in our work,and the absence of the fourth is explained in terms of relative rates of restoration.The mean grain size does indeed increase for UFG (Fig.11b),but not for CG (Fig.10b).The stored energy (Figs.1and 2)Fig.10.Distribution of (a)misorientation and (b)average size of CG copper extruded at different speeds (d 1◦–15◦subgrain size;d 15◦–62.8◦grain size;d 1◦–62.8◦combination of subgrain size and grainsize).Fig.11.Distribution of (a)misorientation and (b)average size of initial UFG and UFG copper extruded at different speeds.and dislocation density (Fig.4)decreases after extrusion of the UFG.However,the misorientation barely changes (Figs.10a and 11a );we explain this by the rate of formation of grains with high angle boundaries forming from low angle boundaries being faster than the rate of rotation of subgrains to higher misorientations;i.e.,grains are leaving the low angle boundary class faster than the mis-orientation is increasing.This argument is supported by the work of Lefevre-Schlick et al.[49],who show that the incubation time for the nucleation of recrystallization by grain boundary bulging in copper is about three times faster than the incubation time for the nucleation of recrystallization by subgrain rotation in the bulk of the grains.Furthermore,the effect of prior restoration on recrys-tallization kinetics is found in work on Al–2.5%Mg [50],where a clear shift of T p to lower temperature is found to be due to prior recrystallization.Thus our hypothesis is shown to be plausible:during continuous heating a decrease in growth rate is offset by enhanced nucleation as a result of restoration mechanisms operating during extrusion,and this serves to reduce both the stored energy and peak tem-perature during subsequent post-extrusion DSC.The remaining question is whether the temperature rise is sufficient during micro-extrusion to allow formation of nuclei/prior recrystallization.For copper,which has a low stacking fault energy,we can reasonably expect dynamic recrystallization if the temperature is high enough during micro-extrusion.Although we cannot measure the temper-ature rise directly because of the complexity of the extrusion die,we can estimate the temperature rise at the different speeds used in this experimental campaign.We must also explain how recrys-tallization comes about in the UFG material and not in the CG.Contributions to the heat rise during extrusion are from (i)defor-mation work,(ii)friction between the billet and the container wall1798 C.F.Gu,C.H.J.Davies/Materials Science and Engineering A527 (2010) 1791–1799 and(iii)friction at die land[51].The latter two of these contribu-tions are primarily at the surface of the extrudate.The temperaturerise during extrusion can be evaluated by summing the compo-nents.The rise in mean temperature( ¯T1)due to thefirst componentcan be calculated as[51]:¯T1=¯ ln ER√3( ×s)(5)where ln ER is the homogeneous strain and the meanflow stress,¯ , is based on the yield stress taken from previous tensile tests[52]; s and are the specific heat(for copper,372J(kg K)−1)and density (8930kg m−3)[53].The temperature rise caused by the second and third compo-nents, ¯T2and ¯T3,are estimated by[51]:¯T2=¯4√3( ×s)V R L B˛(6)¯T3=¯4√×s)V E L D˛(7)where V R is the ram velocity,L B the billet length,˛the thermal diffusivity(for copper,1.174×10−4m2S−1).V E is the exit speed and L D the die land length.The sum of Eqs.(5)–(7)at the various extrusion speeds is the maximum temperature rise possible at the surface of the extrudate. In our case the contributions at the surface(Eqs.(6)and(7))are relatively minor,and the maximum temperature rise during extru-sion of the UFG material ranges from approximately108◦C at the lowest extrusion speed to112◦C at the highest extrusion speed, whereas for the CG material the temperature rise is between45◦C and47◦C.This difference is due to the much largerflow stress of the UFG material.We infer that the initiation of recrystallization during micro-extrusion may be possible for the UFG material[54] but is certainly not possible for the CG.Furthermore,if we take into account the time of the test,at the lower speeds there is suf-ficient time for the heat to dissipate to the die block,whereas at the highest speed with a test duration of12s this is unlikely.We accept that this argument is not rigorous as it neglects changes in theflow stress due to the contrary effects of softening as a result of the rise in temperature and strain hardening.None-the-less this argument does explain the experimental observations and microstructural evolution during micro-extrusion confirms of this explanation.5.ConclusionsWe investigated the thermal stability of UFG and CG copper after micro-extrusion.The thermal stability of UFG copper is improved by extrusion,but declines with extrusion speed and is always poorer than CG copper.The stored energy of CG copper remains approximately constant with extrusion speed and there is no obvi-ous change in peak temperature.In contrast,micro-extrusion of the UFG copper resulted in a reduction of stored energy concur-rent with an unexpected reduction in peak temperature at high extrusion speeds.This is attributed to recrystallization commenc-ing during extrusion.AcknowledgementsThe authors thank Prof.Yuri Estrin(Department of Materials Engineering,Monash University and CSIRO Division of Materials Science and Engineering,Australia)for his insightful discussions. EBSD training from Dr.Tim Williams(MCEM,Australia)is greatly appreciated.The authors are also grateful to Prof.F.J.Humphreys (University of Manchester,United Kingdom)for supplying the VMAP software package and the reviewer for his/her valuable com-ments.References[1]M.Geiger,M.Kleiner,R.Eckstein,N.Tiesler,U.Engel,CIRP Ann.50(2001)445–462.[2]U.Engel,R.Eckstein,J.Mater.Process.Technol.125–126(2002)35–44.[3]N.Krishnan,J.Cao,K.Dohda,J.Manuf.Sci.Eng.129(2007)669–676.[4]A.Rosochowski,W.Presz,L.Olejnik,M.Richert,Int.J.Adv.Manuf.Technol.33(2007)137–146.[5]R.Z.Valiev,N.A.Krasilnikov,N.K.Tsenev,Mater.Sci.Eng.A137(1991)35–40.[6]R.Z.Valiev,R.K.Islamgaliev,I.V.Alexandrov,Progr.Mater.Sci.45(2000)103–189.[7]A.Gholinia,P.B.Prangnell,M.V.Markushev,Acta Mater.48(2000)1115–1130.[8]M.Richert,Q.Liu,N.Hansen,Mater.Sci.Eng.A260(1999)275–283.[9]V.M.Segal,Mater.Sci.Eng.A197(1995)157–164.[10]W.H.Huang,C.Y.Yu,P.W.Kao,C.P.Chang,Mater.Sci.Eng.A366(2004)221–228.[11]A.L.Etter,T.Baudin,C.Rey,R.Penelle,Mater.Charact.56(2006)19–25.[12]O.V.Mishin, D.Juul Jensen,N.Hansen,Mater.Sci.Eng.A342(2003)320–328.[13]Y.M.Wang,E.Ma,M.W.Chen,Appl.Phys.Lett.80(2002)2395.[14]H.S.Kim,Y.Estrin,Mater.Sci.Eng.A410–411(2005)285–289.[15]Y.H.Zhao,Y.T.Zhu,X.Z.Liao,Z.Horita,ngdon,Appl.Phys.Lett.89(2006).[16]D.Jia,Y.M.Wang,K.T.Ramesh,E.Ma,Y.T.Zhu,R.Z.Valiev,Appl.Phys.Lett.79(2001)611.[17]C.C.Koch,Scripta Mater.49(2003)657–662.[18]R.Z.Valiev,I.V.Alexandrov,Y.T.Zhu,T.C.Lowe,J.Mater.Res.17(2002)5–8.[19]K.-T.Park,Y.-S.Kim,J.G.Lee, D.H.Shin,Mater.Sci.Eng.A293(2000)165–172.[20]Y.-H.Zhao,J.F.Bingert,X.-Z.Liao,B.-Z.Cui,K.Han,A.V.Sergueeva,A.K.Mukher-jee,R.Z.Valiev,ngdon,Y.T.Zhu,Adv.Mater.18(2006)2949–2953.[21]Y.Wang,M.Chen,F.Zhou,E.Ma,Nature419(2002)912–914.[22]D.G.Morris,M.A.Mu˜noz-Morris,Acta Mater.50(2002)4047–4060.[23]W.Q.Cao,A.Godfrey,W.Liu,Q.Liu,Mater.Sci.Eng.A360(2003)420–425.[24]Y.H.Zhao,J.F.Bingert,Y.T.Zhu,X.Z.Liao,R.Z.Valiev,Z.Horita,ngdon,Y.Z.Zhou,vernia,Appl.Phys.Lett.92(2008)0819031–0819033.[25]W.Q.Cao,C.F.Gu,E.V.Pereloma,C.H.J.Davies,Mater.Sci.Eng.A492(2008)74–79.[26]T.Ungár,J.Gubicza,G.Ribárik,I.Bertóti,J.Appl.Crystallogr.34(2001)298–310.[27]G.Ribárik,Modeling of diffraction patterns based on microstructural proper-ties,Physics Doctorate School,PhD,Eotvos Lorand University,2008.[28]H.Jazaeri,F.J.Humphreys,Mater.Sci.Forum467–470(2004)63–68.[29]F.J.Humphreys,P.S.Bate,P.J.Hurley,J.Microsc.201(2001)50–58.[30]L.Balogh,J.Gubicza,R.J.Hellmig,Y.Estrin,T.Ungár,Z.Kristallography Suppl.23(2006)381–386.[31]S.Suwas,A.Eberhardt,L.S.Toth,J.J.Fundenberger,T.Grosdidier,Mater.Sci.Forum467–470(2004)1325–1330.[32]J.Gubicza,L.Balogh,R.J.Hellmig,Y.Estrin,T.Ungar,Mater.Sci.Eng.A400–401(2005)334–338.[33]L.Lu,R.Schwaiger,Z.W.Shan,M.Dao,K.Lu,S.Suresh,Acta Mater.53(2005)2169–2179.[34]J.Gubicza,S.Dobatkin,Z.Bakai,N.Q.Chinh,ngdon,Mater.Sci.Forum567–568(2008)181–184.[35]J.Gubicza, C.Nguyen Quang,ngdon,T.Ungar,Microstructure andStrength of Metals Processed by Severe Plastic Deformation,TMS(The Minerals, Metals and Materials Society),Warrendale,PA,USA,2006,pp.231–236.[36]D.Merz,G.Wassermann,Z.Metall.56(1965)516–522.[37]H.Gertel-Kloos,H.G.Brokmeier,H.J.Bunge,Mater.Sci.Forum157–6(1994)685–692.[38]N.Inakazu,Y.Kaneno,H.Inoue,Mater.Sci.Forum157–6(1994)715–720.[39]D.N.Lee,Y.H.Chung,M.C.Shin,Scripta Metall.17(1983)339–342.[40]C.S.Batrrett,T.B.Massalski,Structure of Metals,McGraw Hill,New York,1966,p.543.[41]M.J.Zehetbauer,G.Steiner,E.Schafler,A.Korznikov,E.Korznikova,Deforma-tion Induced Vacancies with Severe Plastic Deformation:Measurements and Modelling,vols.503–504,Trans Tech Publications Ltd.,Fukuoka,Japan,2006, pp.57–64.[42]T.Ungár,E.Schafler,P.Hanák,S.Bernstorff,M.Zehetbauer,Mater.Sci.Eng.A462(2007)398–401.[43]E.Göttler,Philos.Mag.28(1973)1057–1076.[44]M.Wilkens,M.O.Bargouth,Acta Metall.16(1968)465–468.[45]R.Kuˇz el,M.Janeˇc ek,Z.Matˇe j,J.ˇCíˇz ek,M.Dopita,O.Srba,Metall.Mater.Trans.A(2009),doi:10.1007/s11661-009-9895-0.[46]E.Schafler,G.Steiner,E.Korznikova,M.Kerber,M.J.Zehetbauer,Mater.Sci.Eng.A410–411(2005)169–173.[47]F.J.Humphrey,M.Hatherly,Recrystallization and Related Annealing Phenom-ena,Elsevier Science Inc.,NY,1996,p.219.[48]M.J.Starink,A.M.Zahra,Thermochim.Acta292(1997)159–168.。
Gate Oxide Reliability Physical and Computational Models
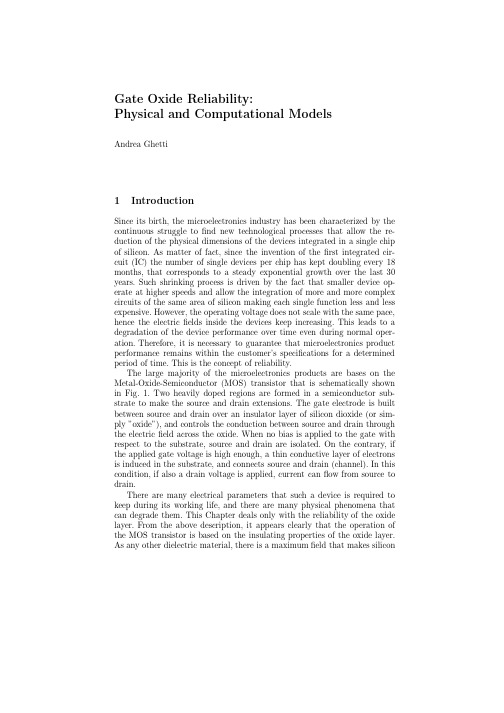
2
Andrea Ghetti
Gate Source in
n+ channel p-substrate
Fig. 1. Schematic structure of a n-MOS transistor
dioxide lose its insulating properties (breakdown) as it is applied (dielectric strength). Obviously, MOS devices operate at lower field. However, if a lower electric field is applied for a long enough time, the oxide slowly degrades (wear out), and eventually breaks down anyway. This phenomenon called time dependent dielectric breakdown (Tddb) is an important parameter for MOS device reliability and it is the object of this Chapter. Usually, time dependent dielectric breakdown is divided in two categories: extrinsic and intrinsic breakdown. Breakdown is defined intrinsic when it is related only to the oxide structure, while it is extrinsic when it is due to defects that can be present because of the many technological steps needed to make an IC. Extrinsic and intrinsic breakdown have different characteristics, and, usually, are characterized in different ways. However, with the continuous reduction of the oxide thickness and increase of the electric field, intrinsic breakdown has become the most likely problem as far as oxide reliability is concerned. In summary, this Chapter is focused on the intrinsic reliability of the gate oxide of MOS devices. Intrinsic oxide reliability is a very complex matter to tackle. There are still many phenomena to be understood. A number of models have been proposed to explain some of the feature of oxide breakdown, but a comprehensive model is still lacking. In this Chapter, we will review the main physical models that have been proposed about intrinsic oxide breakdown. We will try to show pros and cons of each model, possible computational implementation or practical methodology to predict oxide breakdown they enable, and what it is still missing. This Chapter is arranged as follows. Section 2 details the concept of reliability applied to gate oxide in the microelectronics industry, also introducing the essential elements that are common to all reliability models. Sections 3,4,5 describe in details these elements. In particular, Sect. 5 addresses also the issue of the reliability projection provided by the different models. The different types of breakdown are discussed in Sect. 6. Finally, Sect. 7 draws some conclusions.
IODP样品和数据的申请政策IODP_Obligations_Policy_03152012

【精品】外文翻译-使用高光谱成像系统评估猪肉的质量和细脂肪纹路的水2

外文翻译-使用高光谱成像系统评估猪肉的质量和细脂肪纹路的水2使用高光谱成像系统评估猪肉的质量和大理石花纹的水平原文来源:Qiao Jun, Ngadi M O, Wang Ning, Gariepy C, Prasher S O. Pork quality and marbling level assessment using a hyperspectral imaging system. J Food Eng, 2007, 83: 10–16摘要评价猪肉品质通常主观地建立在颜色、大理石花纹和分泌特点的基础之上。
在这项研究中,,为了快速、准确并且客观地评估猪肉的质量,我们对一个基于高光谱成像的方法进行了评价。
此外,大理石花纹水平也被自动确定。
该系统提取出了猪肉样本的光谱特性,获得了猪肉的大理石花纹分布的适当的空间特征,对现有的大理石花纹标准进行了扫描,并且大理石花纹分数指标由共生矩阵来表达。
应用主成分分析(PCA)方法,压缩整个光谱波长的光(430nm–1000nm)到5、10、20主成分(pc),然后将其归属到质量组。
用人工神经网络对这些组进行分类。
结果表明,带红色、坚硬和无渗出物(RFN)的样本与带红色、柔软和有渗出物(RSE)的样本被成功地分组;总准确率为75%-80%。
由前馈神经网络模型归结了修正的类别;其中,5主成分为69%,10主成分为85%。
除了在10主成分里面,二阶矩角(ASM)被成功地用于决定猪肉的大理石花纹分数。
对40个样本加以分类,结果表明样品的大理石花纹分数从3.0到5.0不等。
2007年教育部博士点基金有限公司版权所有。
关键词:高光谱图像;猪肉质量;大理石花纹;主成分分析;聚类分析;神经网络1.前言加拿大是世界上最大的猪肉出口国之一。
随着市场的扩张与分割,为了保持领先地位,加拿大的加工行业需要用来评价猪肉品质的高效技术。
鲜猪肉质量变化极大。
传统上,猪肉质量过去常常根据颜色、纹理(坚实度)和分泌物(滴水损失)分为三个级别。
Mechanical Properties of Cu6Sn5, Cu3Sn, and Ni3Sn4 Intermetallic Compounds Measured by
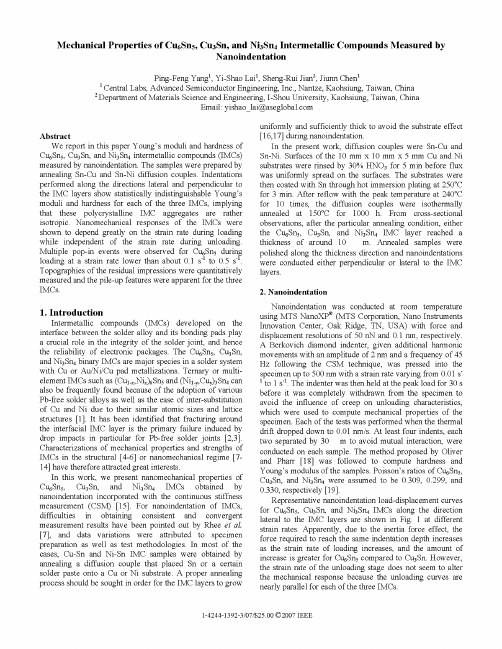
Intermetallic compounds (IMCs) developed on the interface between the solder alloy and its bonding pads play a crucial role in the integrity of the solder joint, and hence the reliability of electronic packages. The Cu6Sn5, Cu3Sn, and Ni3Sn4 binary IMCs are major species in a solder system with Cu or Au/Ni/Cu pad metallizations. Ternary or multielement IMCs such as (Cul-,,Ni,)6Sn5 and (Nil-y,Cuy)3Sn4 can also be frequently found because of the adoption of various Pb-free solder alloys as well as the ease of inter-substitution of Cu and Ni due to their similar atomic sizes and lattice structures [1]. It has been identified that fracturing around the interfacial IMC layer is the primary failure induced by drop impacts in particular for Pb-free solder joints [2,3]. Characterizations of mechanical properties and strengths of IMCs in the structural [4-6] or nanomechanical regime [714] have therefore attracted great interests. In this work, we present nanomechanical properties of Cu6Sn5, Cu3Sn, and Ni3Sn4 IMCs obtained by nanoindentation incorporated with the continuous stiffness measurement (CSM) [15]. For nanoindentation of IMCs, difficulties in obtaining consistent and convergent measurement results have been pointed out by Rhee et al. [7], and data variations were attributed to specimen preparation as well as test methodologies. In most of the cases, Cu-Sn and Ni-Sn IMC samples were obtained by annealing a diffusion couple that placed Sn or a certain solder paste onto a Cu or Ni substrate. A proper annealing process should be sought in order for the IMC layers to grow
Wafer Thinning Techniques for Ultra-thin Wafers - ElectroIQ
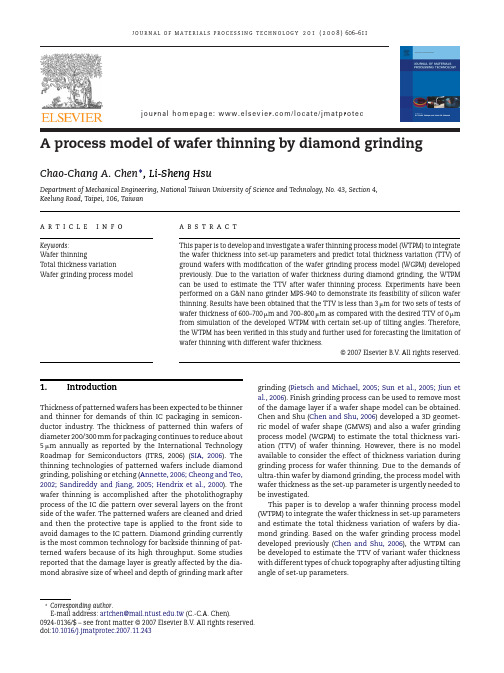
A process model of wafer thinning by diamond grinding
Chao-Chang A. Chen ∗ , Li-Sheng Hsu
Department of Mechanical Engineering, National Taiwan University of Science and Technology, No. 43, Section 4, Keelung Road, Taipei, 106, Taiwan
Total thickness variation Wafer grinding process model
1.
Introduction
Thickness of patterned wafers has been expected to be thinner and thinner for demands of thin IC packaging in semiconductor industry. The thickness of patterned thin wafers of diameter 200/300 mm for packaging continues to reduce about 5 m annually as reported by the International Technology Roadmap for Semiconductors (ITRS, 2006) (SIA, 2006). The thinning technologies of patterned wafers include diamond grinding, polishing or etching (Annette, 2006; Cheong and Teo, 2002; Sandireddy and Jiang, 2005; Hendrix et al., 2000). The wafer thinning is accomplished after the photolithography process of the IC die pattern over several layers on the front side of the wafer. The patterned wafers are cleaned and dried and then the protective tape is applied to the front side to avoid damages to the IC pattern. Diamond grinding currently is the most common technology for backside thinning of patterned wafers because of its high throughput. Some studies reported that the damage layer is greatly affected by the diamond abrasive size of wheel and depth of grinding mark after
RuC高压相变的第一性原理计算外文翻译及原文
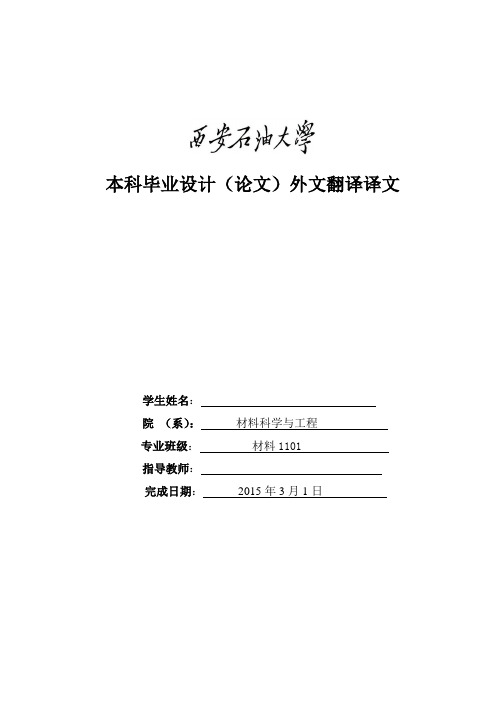
本科毕业设计(论文)外文翻译译文学生姓名:院(系):材料科学与工程专业班级:材料1101指导教师:完成日期:2015年3月1日要求1、外文翻译是毕业设计(论文)的主要内容之一,必须学生独立完成。
2、外文翻译译文内容应与学生的专业或毕业设计(论文)内容相关,不得少于15000印刷符号。
3.外文翻译译文用A4纸打印。
文章标题用3号宋体,章节标题用4号宋体,正文用小4号宋体,20磅行距;页边距上、下、左、右均为2.5cm,左侧装订,装订线0.5cm。
按中文翻译在上,外文原文在下的顺序装订。
4、年月日等的填写,用阿拉伯数字书写,要符合《关于出版物上数字用法的试行规定》,如“2005年2月26日”。
5、所有签名必须手写,不得打印。
RuC高压相变的第一性原理计算First-principle calculations of high-pressure phasetransformations in RuC作者:Jian Hao, Xiao Tang, Wenjing Li, Yinwei Li起止页码:46004-p1~p5出版日期(期刊号):EPL, 105 (2014) 46004,2014年2月27日出版单位:IOP, EPL (Europhysics Letters)摘要- 使用第一原理计算在高压下RuC的结构稳定性。
结果表明,在9.3GPa的压力下,RuC从ZB型(闪锌矿型)结构转变为空间群为I4mm的四面体结构。
通过RuC5金字塔构造的I4mm结构的稳定性达26GPa,在更高压力下,则更有利成为WC型结构。
观察到伴随ZB型→ I4mm → WC型的相序,配位数增加从4至5,然后至6。
能带结构的计算表明,ZB型相是半导体,而I4mm和WC型相是金属。
此外,对所有三个阶段的RuC的机械特性进行了讨论。
简介-经压缩,由于原子间相互作用的变化和电子密度的再分配,化合物通常经历若干次相变。
结构的变化也因此可以引起物理性质的剧烈变化[1]。
固态电解质的理论计算与实验研究进展述评
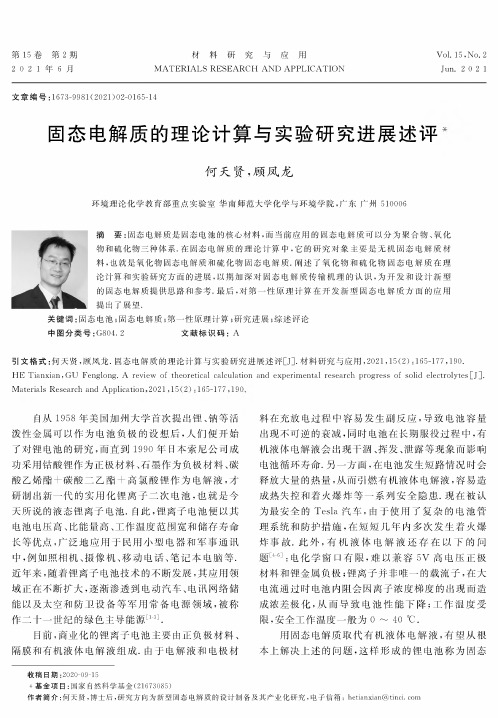
的固态电解质提供思路和参考.最后,对第一性原理计算在开发新型固态电解质方面的应用
提出了展望. 关键词:固态电池;固态电解质;第一性原理计算;研究进展;综述评论
中图分类号:G804. 2
文献标识码:A
引文格式:何天贤,顾凤龙.固态电解质的理论计算与实验研究进展述评(J).材料研究与应用,2021,15(2):165-177,190. HE Tianxian, GU Fenglong. A review of theoretical calculation and experimental research progress of solid electrolytes (J]. MaterialsResearchand Application 2021 15 2):165-177 190
一定的条件(图1".
离子电导率
室温离子电导达到1(T'S・cm"以上, 这是保证锂离子电池快速充放电的 基本条件
固态电解质 评判标准
力学性能
高力学强度的固态电解质能抵制晶枝 的生长,高机械强度易于大规模生产
离子迁移数
理想状态为1,迁移数过低时阴离子会 在电极表面富集,导致电池极化加剧, 电阻增大
电极材料兼容性 保证良好的界面接触,其单位面积的 界面电阻应当尽可能小
制造成本 制造成本低廉,以利于市场开发
热力学/化学/电化学稳定性 为保证电池在较宽的温度范围内使用,固态电解质必须有 足够的热力学稳定性;保证在正负极之间不会发生副反应; 电化学窗口可以达到4.5 V以上
图1固态电解质的评判标准 Fig. 1 Judgment criteria for solid electrolyte
多样化的要求使得探索固态电解质是一个持续 性的话题.从1957年开始人们就在不断研究固态电 解质,但是至今仍然没有一种称得上是完美的固态 电解质.目前,应用的固态电解质有三种体系,分别
BS 970-1 - 1996 General Inspection And Testing Procedures And Specific Requirements For Carbon, Carb
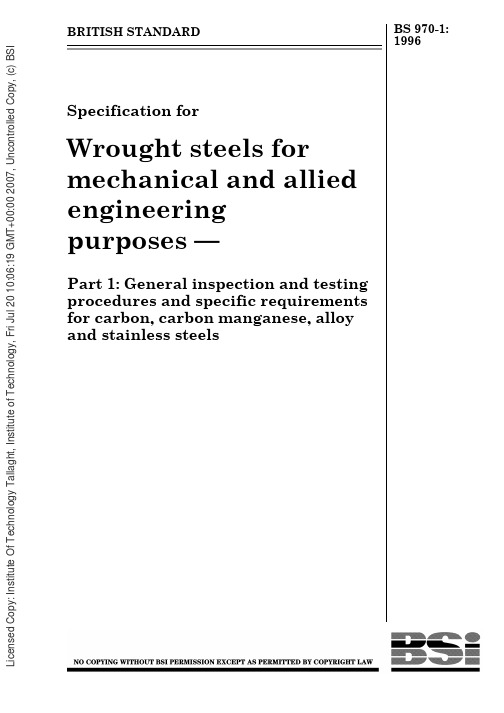
16
Section 5. Specific requirements for stainless and heat resisting steels
24
Section 6. Specific requirements for sizes and tolerances
6.1 Sizes
27
6.2 Mass/unit length tolerances
8
1.14 Selection and preparation of material for mechanical testing
8
1.15 Frequency of other tests
9
1.16 Test methods and test results
10
1.17 Retests
11
1.18 Inspection
ISBN 0 580 25120 9
Licensed Copy: Institute Of Technology Tallaght, Institute of Technology, Fri Jul 20 10:06:19 GMT+00:00 2007, Uncontrolled Copy, (c) BSI
© BSI 10-1998
Contents
BS 970-1:1996
Committees responsible Foreword
Page Inside front cover
iii
Section 1. General inspection and testing procedure
1.1 Scope
1
1.2 References
1
photocatalysis
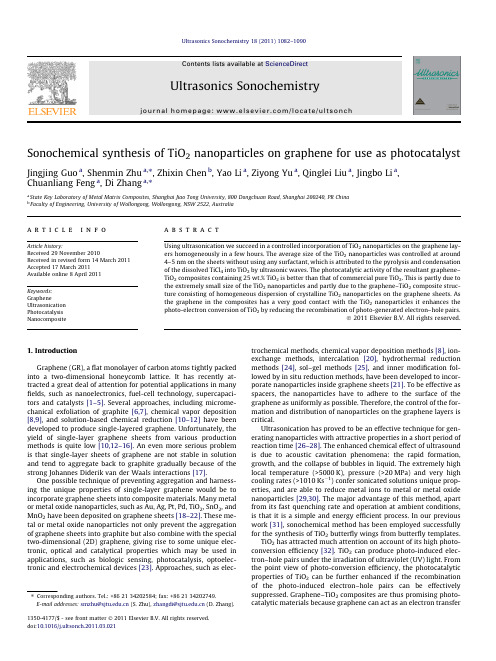
Sonochemical synthesis of TiO 2nanoparticles on graphene for use as photocatalystJingjing Guo a ,Shenmin Zhu a ,⇑,Zhixin Chen b ,Yao Li a ,Ziyong Yu a ,Qinglei Liu a ,Jingbo Li a ,Chuanliang Feng a ,Di Zhang a ,⇑a State Key Laboratory of Metal Matrix Composites,Shanghai Jiao Tong University,800Dongchuan Road,Shanghai 200240,PR China bFaculty of Engineering,University of Wollongong,Wollongong,NSW 2522,Australiaa r t i c l e i n f o Article history:Received 29November 2010Received in revised form 14March 2011Accepted 17March 2011Available online 8April 2011Keywords:GrapheneUltrasonication Photocatalysis Nanocompositea b s t r a c tUsing ultrasonication we succeed in a controlled incorporation of TiO 2nanoparticles on the graphene lay-ers homogeneously in a few hours.The average size of the TiO 2nanoparticles was controlled at around 4–5nm on the sheets without using any surfactant,which is attributed to the pyrolysis and condensation of the dissolved TiCl 4into TiO 2by ultrasonic waves.The photocatalytic activity of the resultant graphene–TiO 2composites containing 25wt.%TiO 2is better than that of commercial pure TiO 2.This is partly due to the extremely small size of the TiO 2nanoparticles and partly due to the graphene–TiO 2composite struc-ture consisting of homogeneous dispersion of crystalline TiO 2nanoparticles on the graphene sheets.As the graphene in the composites has a very good contact with the TiO 2nanoparticles it enhances the photo-electron conversion of TiO 2by reducing the recombination of photo-generated electron–hole pairs.Ó2011Elsevier B.V.All rights reserved.1.IntroductionGraphene (GR),a flat monolayer of carbon atoms tightly packed into a two-dimensional honeycomb lattice.It has recently at-tracted a great deal of attention for potential applications in many fields,such as nanoelectronics,fuel-cell technology,supercapaci-tors and catalysts [1–5].Several approaches,including microme-chanical exfoliation of graphite [6,7],chemical vapor deposition [8,9],and solution-based chemical reduction [10–12]have been developed to produce single-layered graphene.Unfortunately,the yield of single-layer graphene sheets from various production methods is quite low [10,12–16].An even more serious problem is that single-layer sheets of graphene are not stable in solution and tend to aggregate back to graphite gradually because of the strong Johannes Diderik van der Waals interactions [17].One possible technique of preventing aggregation and harness-ing the unique properties of single-layer graphene would be to incorporate graphene sheets into composite materials.Many metal or metal oxide nanoparticles,such as Au,Ag,Pt,Pd,TiO 2,SnO 2,and MnO 2have been deposited on graphene sheets [18–22].These me-tal or metal oxide nanoparticles not only prevent the aggregation of graphene sheets into graphite but also combine with the special two-dimensional (2D)graphene,giving rise to some unique elec-tronic,optical and catalytical properties which may be used in applications,such as biologic sensing,photocatalysis,optoelec-tronic and electrochemical devices [23].Approaches,such as elec-trochemical methods,chemical vapor deposition methods [8],ion-exchange methods,intercalation [20],hydrothermal reduction methods [24],sol–gel methods [25],and inner modification fol-lowed by in situ reduction methods,have been developed to incor-porate nanoparticles inside graphene sheets [21].To be effective as spacers,the nanoparticles have to adhere to the surface of the graphene as uniformly as possible.Therefore,the control of the for-mation and distribution of nanoparticles on the graphene layers is critical.Ultrasonication has proved to be an effective technique for gen-erating nanoparticles with attractive properties in a short period of reaction time [26–28].The enhanced chemical effect of ultrasound is due to acoustic cavitation phenomena:the rapid formation,growth,and the collapse of bubbles in liquid.The extremely high local temperature (>5000K),pressure (>20MPa)and very high cooling rates (>1010Ks À1)confer sonicated solutions unique prop-erties,and are able to reduce metal ions to metal or metal oxide nanoparticles [29,30].The major advantage of this method,apart from its fast quenching rate and operation at ambient conditions,is that it is a simple and energy efficient process.In our previous work [31],sonochemical method has been employed successfully for the synthesis of TiO 2butterfly wings from butterfly templates.TiO 2has attracted much attention on account of its high photo-conversion efficiency [32].TiO 2can produce photo-induced elec-tron–hole pairs under the irradiation of ultraviolet (UV)light.From the point view of photo-conversion efficiency,the photocatalytic properties of TiO 2can be further enhanced if the recombination of the photo-induced electron–hole pairs can be effectively suppressed.Graphene–TiO 2composites are thus promising photo-catalytic materials because graphene can act as an electron transfer1350-4177/$-see front matter Ó2011Elsevier B.V.All rights reserved.doi:10.1016/j.ultsonch.2011.03.021Corresponding authors.Tel.:+862134202584;fax:+862134202749.E-mail addresses:smzhu@ (S.Zhu),zhangdi@ (D.Zhang).channel thus reduces the recombination of the photo-generated electron holes,and leads to improved photo-conversion efficiency [33,34].It was reported that a mixture of commercially pure TiO2 (P25)powder and graphene showed a relatively high photocata-lytic property[24].However,the particle size of P25was relative large and in micrometers and thus the photocatalytic property can be further improved if the size of TiO2is reduced to nanome-ters.In fact,TiO2particle size needs to be reduced down to nanometers in order to improve the photocatalytic activity for liquid-phase oxidation.In this paper,we report a sonochemical method that embeds TiO2nanoparticles into graphene oxide(GO)nanosheets homoge-neously without functionalizing the surface with a surfactant. Graphene–TiO2composite(GR–TiO2)was obtained by chemical reduction of the graphene oxide–TiO2composite(GO–TiO2).The photocatalytic properties of the obtained GR–TiO2composites were investigated by measuring the photo-degradation of methy-lene blue under UV-light,which demonstrated a high photocata-lytic performance for the obtained composites.2.Experimental2.1.Preparation of GOGO was prepared from natural graphite(crystalline,300mesh, Alfa Aesar)by a modified Hummers method[35].The detailed pro-cessing is described as below:2g of graphite and1g of NaNO3 were mixed with46ml of H2SO4(98%)in a250ml wide necked bottle placed in an ice bath.Then,the mixture was stirred for 30min.While maintaining magnetic vigorous stirring,a certain amount of KMnO4(6g)was added to the suspension carefully. After that,the ice bath was replaced by an oil bath,and the mixture was stirred at15°C for2h.As the reaction progressed,the mixture gradually became pasty,and the color turned into light brownish. The next step was to increase the reaction temperature to40°C and keep for another1h.Then,92ml of H2O was slowly added to the pasty mixture with vigorous agitation.The reaction temper-ature was rapidly increased to98°C with effervescence,and the color changed to yellow.Finally,30ml of5%H2O2was added to the mixture and allowing the high-temperature reaction to go on for1h.For purification,the mixture was rinsed and centrifugated with5%HCl and deionized water for several times.Afterfiltration and drying under vacuum at60°C,GO was obtained as a gray powder.2.2.Preparation of GO–TiO2GO–TiO2composite was synthesized by sonochemical reaction of TiCl4in the presence of the GO.The detailed process is described as follows:firstly,TiO2precursors were prepared with the molar ratios of ethanol:H2O:TiCl4=35:11:1,then,0.25g of the GO was added and stirred for0.5h at ambient temperature,andfinally the suspension was sonicated at room temperature for3h using a high-intensity ultrasonic probe(Ti horn,20kHz,100W/cm2). The resulting composite was recovered by centrifugation and rins-ing with ethanol solvent several times,then dried under vacuum at 60°C.The obtained sample is called GO–TiO2.2.3.Preparation of GR–TiO2Chemical conversion of GO–TiO2to GR–TiO2was carried out by using a reduction method[36].In a typical reduction experiment, 0.7g of GO–TiO2powder was dispersed in180ml of deionized water and the mixture was sonicated for1h.Next,24ml of hydra-zine(85%)was added into the mixture under magnetic stirring and stirred for24h at50°C.GR–TiO2composite was obtained byfiltra-tion and drying under vacuum at60°C.After calcination at450°C for3h under nitrogen,crystallized GR–TiO2composite(GR–TiO2–T)was obtained.2.4.Preparation of grapheneGraphene was obtained by chemical conversion of GO according to the literature[36].In a typical reduction experiment,0.5g of GO powder was dispersed in150ml of deionized water and the mix-ture was sonicated for1h.Next,18ml of hydrazine(85%)was added under magnetic stirring,and the mixture was continuously stirred at50°C for24h.Finally,black graphene powder was obtained byfiltration and drying under vacuum at60°C.2.5.CharacterizationThe synthesized samples were characterized by X-ray diffrac-tion(XRD)using a RigakuD/max2550VL/PC system operated at 40kV and40mA with Cu K a radiation(k=1.5406Å),at a scan rate of5°minÀ1and a step size of0.050°in2h.Nitrogen adsorption measurements at77K were performed using an ASAP2020volu-metric adsorption analyzer,after the samples had been outgassed for8h in the degas port of the adsorption apparatus.Field-emis-sion scanning electron microscopy(FE-SEM)was performed on a JEOL JSM-6360LVfield emission microscope at an accelerating voltage of15kV.Transmission electron microscopy(TEM)and en-ergy-dispersive X-ray measurements(EDX)were carried out on a JEOL2010microscope at200kV.TEM specimens were prepared by grinding the synthesized samples into powder with a mortar and pestle and the powder was dispersed in pure ethanol and picked up with holey carbon supportingfilms on copper grids.Fou-rier transform-infrared measurements(FT-IR)were recorded on KBr pellets with a PE Paragon1000spectrophotometer.Thermal gravimetric analysis(TGA)was conducted on a PE TGA-7instru-ment with a heating rate of20°C/min.X-ray photoelectron spectra (XPS)were collected on a physical electronics PHI5400using Mg K a radiation as X-ray source.All the spectra were corrected with the C1s(285.0eV)band.Diffuse reflectance electronic spectra were measured with a Perkin–Elmer330spectrophotometer equipped with a60mm Hitachi integrating sphere accessory.2.6.Photocatalytic experiments30mg of catalyst(GR,TiO2,and GR–TiO2–T)was dispersed in a 40ml0.02g/L aqueous solution of methylene blue dye in a quartz test cell.The reaction system was kept saturated with oxygen by purging a slow stream of oxygen at ambient temperature.Before irradiation,the reactor was stirred constantly in the presence of oxygen in dark for30min,and then irradiated by four UV lamps (6W each)at room temperature.The photocatalytic activity was evaluated on the basis of decrease of the absorbance band of the methylene blue at665nm recorded at a regular time interval fol-lowing the UV illumination.The absorbance measurement of the reaction solution was taken after separating the TiO2from the reaction system by centrifugation.3.Results and discussion3.1.CharacterizationsWide-angle XRD patterns of the pure TiO2,GR,GR–TiO2and GR–TiO2–T are given in Fig.1.The very broad peak at2h=25.5°of GR–TiO2indicates that TiO2in the sample is largely of amor-phous in nature.After the calcination at450°C,this peak atJ.Guo et al./Ultrasonics Sonochemistry18(2011)1082–109010832h=25.5°becomes relatively narrower and resolved,indicating that nanocrystalline TiO2formed on the graphene.However,the peak is still too broad to be used to estimate the size of crystallites with Scherrer equation[37].Nevertheless the broad peak suggests that growth of the nanocrystalline TiO2on the graphene was very limited during the calcinations.The loading of the TiO2nanoparticles on the graphene was determined by performing TGA in air by heating up from40to 900°C(Fig.2).A significant mass loss was observed at tempera-tures from510to700°C for both GR and GR–TiO2–T,due to the destruction of the carbon skeleton(carbonyl/double bond)of graphene.The weight loss of GR–TiO2–T was stabilized at75%atthe graphene sheets in the GR–TiO2–T composite exhibited nano-scale textures,indicative of a much rougher surface(Fig.3b).At a high magnification(Fig.3d),we can clearly see the uniform disper-sion of TiO2nanoparticles on both the graphene surface and the interlayers.There is no agglomeration in the composite as shown in the SEM image of the GR–TiO2sheet(Fig.3f).Fig.3f also shows that the TiO2particle size was in nanometers which will be further characterized by using TEM.Fig.4shows TEM images of TiO2loaded on the graphene layers before(GR–TiO2)and after the calcination at450°C for3h(GR–TiO2–T).For the as-synthesized GR–TiO2(Fig.4a),there are plenty of wrinkles(in circles and at the edge)on the clean sheet owing to the2D nature of the sheet.The edges of the sheets are folded and give the appearance of ribbons.High-magnification TEM micro-graphs(Fig.4b and c)reveal no discrete and well defined nanocrys-talline TiO2particles on the graphene sheets.However EDX(see Supporting Information,Fig.s1)shows the presence of Ti,O and C in the sample.The corresponding selected area electron diffrac-tion pattern(SAED)of the composite shows that diffraction spots of the graphite superimposed with the diffraction rings of TiO2 nanoparticles.After the calcination at450°C for3h,discrete and well defined TiO2fully crystallized particles were uniformly scat-tered on the sheets as shown in Fig.4d and the particle size is around4–5nm from the high resolution image(Fig.4f).This obser-vation is consistent with the wide angle XRD results presented ear-lier(Fig.1).The inset in Fig.4f is the SAED of GR–TiO2–T,and it can be seen that the sample has a clear sixfold pattern of the graphene and the diffraction rings of the TiO2nanoparticles.The existence of elements Ti,O and C was detected by EDX analysis(see Supporting Information Fig.s2).As demonstrated in Fig.4e,the measured lat-tice-fringe spacing of0.34nm in the ribbons corresponds to the (002)of the graphene sheets,where the crystal lattice of TiO2 nanoparticles is also resolved.From Fig.4f the measured lattice fringe spacing of0.355nm in GR–TiO2–T composites corresponds to the(101)of anatase TiO2(JCPDS21-1272).No agglomeration of the TiO2particles was observed in this sample,which is usually observed in samples prepared by other methods[20,24].The TEM images shown in Fig.4confirm that a uniform disper-sion of TiO2nanoparticles on the graphene sheets have been achieved under sonochemical reactions without the use of any sur-factant.Which means ultrasound is very effective in dispersing TiO2nanoparticles on graphene layers.In order to understand the texture of the composites,nitrogen isothermal adsorption technique was employed to investigate the ultrasonic irradiation effect on the pore structure as well as to determine the location of the TiO2in graphene(Fig.5).The shape of the isotherm for GR–TiO2–T was similar to that of the parent GR,indicating the presence of open pores.It has a specific surface area of49m2gÀ1according to the BET(Brunauer,Emmett and Tell-er)analysis.The P/P0position of the inflection points is related to the pore size in mesopore range,and the sharpness of these steps indicates the uniformity[38].As the relative pressure increases, both the isotherms of GR and GR–TiO2–T exhibit sharp inflections in the P/P0in the range0.5–0.6,a characteristic of capillary con-densation within a uniform of interlayer spacing.Interestingly, the space layer distribution of the starting GR centered at2.6nm but disappeared when the TiO2was loaded,suggesting the possi-bility that titanium oxide formedfirst in this interlayer spacing. As a result,the space layer distribution of GR–TiO2–T is more uni-form than that of the pure GR from pore size distributions shown in Fig.5(inset).The metal oxide nanoparticles increased the dis-tance between the GR sheets to several nanometers,thereby mak-ing the both faces of the graphene accessible.The light-absorbance property of the samples was studied with a UV–vis spectrophotometer and the measured UV–visible diffuse reflectance electronic spectra are shown in Fig.6.The absorption 18(2011)1082–1090bands of GR–TiO2–T appeared to be fairly different from that of P25.Because the appearance of TiO2is white whereas the GR–TiO2–T is black,the Kubelka–Munk theory could not apply to this sample with such a strong adsorption.Therefore,the quantitative consideration is void between TiO2and GR–TiO2–T.However,a broad and strong absorption in the visible light region was ob-served for GR–TiO2–T due to the presence of75wt.%GR in the GR–TiO2–T composite which acts as a‘‘dyade’’structure[39].This enhanced light-harvesting intensity of GR–TiO2–T could be possi-bly explained by the formation of the chemical bonding between TiO2and GR,i.e.a Ti–O–C bond which favors charge transfer upon light excitation[40].The formation of the Ti–O–C bond was confirmed by FT-IR spec-troscopy as shown in Fig.7.GO depicts a strong OH peak at 3389.4cmÀ1and other C–O functionalities such as COOH (1726.6cmÀ1)and COC/COH(1383.3–1055.3cmÀ1)are clearly vis-ible.The spectrum also shows a C@C peak at1617.5cmÀ1corre-sponding to the remaining sp2character.After the hydrazine reduction,the characteristic absorption peaks(at1726.6,1383.3–1055.3,and591.2cmÀ1)of GO disappeared due to the reduction of oxygenous groups from the GO.As for GR–TiO2–T,the broad absorptions at low frequencies(below1000cmÀ1)were ascribed to the vibration of Ti–O–Ti bond(671.3,683.9cmÀ1)and Ti–O–C bond(780.2cmÀ1)which was not observed in the spectrum of GR.This demonstrates that the TiO2nanoparticles were strong chemically bonded on the graphene sheets,which was facilitated by the sonochemical reaction.Further it was found that the peak of the stretching vibration C-OH at3419.7cmÀ1of GR shifted to a higher wave number of3443.8cmÀ1for GR–TiO2–T,which could be explained by the influence of the formation of Ti–O–C bond. Similar to that of GR,the skeletal vibration of the GR sheets at 1569.6cmÀ1was also clearly observed for GR–TiO2–T,illustrating the formation of GR–TiO2–T composites.Due to the Ti–O–C bond the TiO2nanoparticles in GR–TiO2–T did not grow much upon cal-cinations,i.e.the host graphene sheet prevented the nanocrystals from sintering.Complicated titanium coordination states in GR–TiO2–T,were revealed from the XPS results.Fig.8a shows the Ti2p XPS spectra of GR–TiO2–T,GR and GO.As expected,there are two peaks,Ti2p3/2 centered at458.5eV and Ti2p1/2at464.2eV in the XPS spectrum of GR–TiO2–T,which were not detected in the spectra of GR and GO.Fig.8b shows the XPS spectra of GR–TiO2–T,GO and GR in the binding energy range between280and294eV(C1s).The two peaks at287and285eV of GO indicate a considerable degree of oxidation of the graphite.The binding energy of285eV is due to non-oxygenated ring C(284.3eV),and287eV is due to the C in C–O bonds(285.2eV),the carbonyl C(C@O,287.4eV)and carboxyl C (COOH,289.1eV)respectively[41].Both the C1s in the XPS spec-tra of GR and GR–TiO2–T exhibit only a single strong peak at 284.3eV which is attributed to elemental carbon,with aweak Fig.3.FE-SEM images of GR(a,c,e)and GR–TiO2–T(b,d,f)in different magnifications.Fig.6.Diffuse reflectance electronic spectra of pure TiO2and GR–TiO2–T.XPS spectra of GO,GR and GR–TiO2–T,(b)the C1s XPS spectra of GO,GR and GR–TiO2–T,(c)the survey XPS spectra of GO,electrostatic binding,or through charge-transfermight become attached to the carboxylto TiO2takes place on the surface of theHowever,the number of ionizable carboxylenough to complex the metal cationsoxide particles formed,resulting in lessparticles.Generally,surface functionaliza-be effective for homogeneous dispersionSB12is generally used to control the sizeprevent aggregation[42].Here in our pro-nanoparticles were formed on the surface of graph-modification or additional agent.The unique ultrasonication may have effects on the formation aggregation of the TiCl4on the surface of the GO sheets can be sig-nificantly retarded by the collapse of small bubbles,resulting in a layer coating,a few nanometers thick,on the GO surface,which is consistent with the disappearance of layer spacing at2.6nm from the BET analysis.As a result,uniform dispersion offine TiO2 forms in situ without any surface functionalization.The one-step formation of the TiO2-graphene composites under ultrasonic irra-diation illustrates the simplicity and efficiency of the sonochemical approach as compared with the commonly used sol–gel’s proce-dure which is generally complex and very time-consuming.3.3.Photocatalytic measurementsThe photocatalytic activities of GR–TiO2–T were measured by the photo-degradation of methylene blue as model reaction under UV-light(6400nm),and the results are shown in Fig.10.For a comparison,the photocatalytic activities of commercial TiO2and pure GR were also measured under the same condition.In order to exclude the influence of adsorption process,a time of60min was allowed to achieve adsorption equilibrium before the photo-catalytic reaction.Fig.10shows that the concentration of methy-lene blue decreases fast with irradiation time both for TiO2and GR–TiO2–T,but almost no concentration change was observed for the pure GR,illustrating that the photocatalytic reaction is related to the existence of active sites(here TiO2).The pseudo-first-order reaction was observed for the both TiO2and GR–TiO2–T(Fig.11). For GR–TiO2–T,the k value at0.0139is higher than that of P25 at0.0054;a high k value is well known to have a high photocata-lytic activity.Photocatalytic efficiency can be affected by three things:crystalline phase,surface area and hierarchical structures. It is also well known that the activity is proportional to the surface area accessible to the liquid.A large surface area could adsorb sig-nificant amounts of water thus hydroxyl groups which can react with photo excited holes and produce hydroxyl radicals which are powerful oxidants in degrading organics.In this investigation, the surface area of GR–TiO2–T was almost the same as that of TiO2but the former showed much higher photocatalytic activity than the latter.This may be explained by the crystalline TiO2nano-particles prepared by sonochemical and calcinations approach, which enhance the activity by facilitating the access to the reactive sites of TiO2.Further,the graphene in the composite can act as an electron transfer channel to reduce the recombination of the photo-generated electron holes,and lead to improved photo-con-version efficiency[33,34].It should be noted that the weight ratio of the TiO2in GR–TiO2–T composite is only25wt.%,so the real9.The proposed TiO2nanoparticles formation mechanism on the graphene sheets by sonochemical method.Fig.10.Photo-degradation of methylene blue solution under UV-light over GR,TiOphotocatalytic activity of the TiO 2nanoparticles should be even higher than commercial pure TiO 2.4.ConclusionsUsing ultrasonication we succeed in controlled incorporation of TiO 2on the graphene layers homogeneously in a few hours.The average particle size of the TiO 2was controlled at around 4–5nm on the sheets without using any surfactant,which is attrib-uted to the pyrolysis and condensation of the dissolved TiCl 4into TiO 2by ultrasonic waves.The synthesis process is simple and effi-cient.The photocatalytic performance of GR–TiO 2–T containing 25wt.%TiO 2is better than commercial pure TiO 2.The much im-proved photocatalytic activity of GR–TiO 2–T,prepared by sono-chemical method,is attributed to the graphene–TiO 2composite structure consisting of very small and homogeneous dispersion of crystal TiO 2on the graphene sheets.In this case the photocata-lytic activity of the TiO 2is enhanced not only by the fine size but also by the graphene which reduces the recombination of photo-generated electron–hole pairs.Thus,such integration of 2D supports with large surface areas,and the highly dispersed nanoparticles,can be an exciting material for use in future nano-technology.These results and other related data demonstrate that this sonochemical method may also be extended to synthesize other metal oxides on graphene sheets,with high performance in many potential applications,such as optical,electrical,catalysis,sensors,and energy conversion devices and so on.AcknowledgmentsThe authors gratefully acknowledge the financial support of this research by the National Science Foundation of China (Nos.51072117,50772067),Shanghai Science and Technology Commit-tee (Nos.06PJ14063,07DJ14001),and Sino-French Project of MOST of China (No.2009DFA52410).We also thank SJTU Instrument Analysis Center for the measurements.Appendix A.Supplementary dataSupplementary data associated with this article can be found,in the online version,at doi:10.1016/j.ultsonch.2011.03.021.References[1]A.K.Geim,K.S.Novoselov,The rise of graphene,Nat.Mater.6(2007)183–191.[2]S.Watcharotone,D.A.Dikin,S.Stankovich,R.Piner,I.Jung,G.H.B.Dommett,G.Evmenenko,S.E.Wu,S.F.Chen,C.P.Liu,S.T.Nguyen,R.S.Ruoff,Graphene–silica composite thin films as transparent conductors,Nano Lett.7(2007)1888–1892.[3]X.Wang,L.J.Zhi,K.Mullen,Transparent,conductive graphene electrodes fordye-sensitized solar cells,Nano Lett.8(2008)323–327.[4]J.C.Meyer,A.K.Geim,M.I.Katsnelson,K.S.Novoselov,T.J.Booth,S.Roth,Thestructure of suspended graphene sheets,Nature 446(2007)60–63.[5]M.Ishigami,J.H.Chen,W.G.Cullen,M.S.Fuhrer, E.D.Williams,Atomicstructure of graphene on SiO 2,Nano Lett.7(2007)1643–1648.[6]K.S.Novoselov,A.K.Geim,S.V.Morozov,D.Jiang,Y.Zhang,S.V.Dubonos,I.V.Grigorieva,A.A.Firsov,Electric field effect in atomically thin carbon films,Science 306(2004)666–669.[7]A.Dato,V.Radmilovic,Z.H.Lee,J.Phillips,M.Frenklach,Substrate-free gas-phase synthesis of graphene sheets,Nano Lett.8(2008)2012–2016.[8]K.S.Kim,Y.Zhao,H.Jang,S.Y.Lee,J.M.Kim,K.S.Kim,J.H.Ahn,P.Kim,J.Y.Choi,B.H.Hong,Large-scale pattern growth of graphene films for stretchable transparent electrodes,Nature 457(2009)706–710.[9]A.Reina,X.T.Jia,J.Ho,D.Nezich,H.B.Son,V.Bulovic,M.S.Dresselhaus,J.Kong,Large area,few-layer graphene films on arbitrary substrates by chemical vapor deposition,Nano Lett.9(2009)30–35.[10]D.Li,M.B.Muller,S.Gilje,R.B.Kaner,G.G.Wallace,Processableaqueous dispersions of graphene nanosheets,Nat.Nanotechnol.3(2008)101–105.[11]S.Park,R.S.Ruoff,Chemical methods for the production of graphenes,Nat.Nanotechnol.4(2009)217–224.[12]S.Stankovich,D.A.Dikin,R.D.Piner,K.A.Kohlhaas,A.Kleinhammes,Y.Jia,Y.Wu,S.T.Nguyen,R.S.Ruoff,Synthesis of graphene-based nanosheets via chemical reduction of exfoliated graphite oxide,Carbon 45(2007)1558–1565.[13]Y.Hernandez,V.Nicolosi,M.Lotya,F.M.Blighe,Z.Y.Sun,S.De,I.T.McGovern,B.Holland,M.Byrne,Y.K.Gun’ko,J.J.Boland,P.Niraj,G.Duesberg,S.Krishnamurthy,R.Goodhue,J.Hutchison,V.Scardaci, A.C.Ferrari,J.N.Coleman,High-yield production of graphene by liquid-phase exfoliation of graphite,Nat.Nanotechnol.3(2008)563–568.[14]hravathi,M.Rajamathi,Chemically modified graphene sheets producedby the solvothermal reduction of colloidal dispersions of graphite oxide,Carbon 46(2008)1994–1998.[15]K.S.Novoselov, A.K.Geim,S.V.Morozov, D.Jiang,M.I.Katsnelson,I.V.Grigorieva,S.V.Dubonos,A.A.Firsov,Two-dimensional gas of massless Dirac fermions in graphene,Nature 438(2005)197–200.[16]P.W.Sutter,J.I.Flege,E.A.Sutter,Epitaxial graphene on ruthenium,Nat.Mater.7(2008)406–411.[17]D.F.Leigh,C.Norenberg,D.Cattaneo,J.H.G.Owen,K.Porfyrakis,A.L.Bassi,A.Ardavan,G.A.D.Briggs,Self-assembly of trimetallic nitride template fullerenes on surfaces studied by STM,Surf.Sci.601(2007)2750–2755.[18]R.Muszynski,B.Seger,P.V.Kamat,Decorating graphene sheets with goldnanoparticles,J.Phys.Chem.C 112(2008)5263–5266.[19]C.Xu,X.Wang,J.W.Zhu,Graphene-metal particle nanocomposites,J.Phys.Chem.C 112(2008)19841–19845.[20]G.Williams, B.Seger,P.V.Kamat,TiO 2–graphene nanocomposites UV-assisted photocatalytic reduction of graphene oxide,ACS Nano 2(2008)1487–1491.[21]T.Cassagneau,J.H.Fendler,Preparation and layer-by-layer self-assembly ofsilver nanoparticles capped by graphite oxide nanosheets,J.Phys.Chem.B 103(1999)1789–1793.[22]Y.C.Si, E.T.Samulski,Exfoliated graphene separated by platinumnanoparticles,Chem.Mater.20(2008)6792–6797.[23]C.Xu,X.Wang,Fabrication of flexible metal-nanoparticte film using grapheneoxide sheets as substrates,Small 5(2009)2212–2217.[24]H.Zhang,X.J.Lv,Y.M.Li,Y.Wang,J.H.Li,P25–graphene composite as a highperformance photocatalyst,ACS Nano 4(2010)380–386.[25]X.Y.Zhang,H.P.Li,X.L.Cui,Y.H.Lin,Graphene/TiO 2nanocomposites:synthesis,characterization and application in hydrogen evolution from water photocatalytic splitting,J.Mater.Chem.20(2010)2801–2806.[26]J.C.Yu,J.G.Yu,W.K.Ho,L.Z.Zhang,Preparation of highly photocatalytic activenano-sized TiO 2particles via ultrasonic irradiation,mun.(2001)1942–1943.[27]G.A.Tai,W.L.Guo,Sonochemistry-assisted microwave synthesis and opticalstudy of single-crystalline US nanoflowers,Ultrason.Sonochem.15(2008)350–356.[28]A.Gedanken,Using sonochemistry for the fabrication of nanomaterials,Ultrason.Sonochem.11(2004)47–55.[29]A.Gedanken,X.H.Tang,Y.Q.Wang,N.Perkas,Y.Koltypin,ndau,L.Vradman,M.Herskowitz,Using sonochemical methods for the preparation of mesoporous materials and for the deposition of catalysts into the mesopores,Chem.-Eur.J.7(2001)4546–4552.[30]W.Chen,W.P.Cai,C.H.Liang,L.D.Zhang,Synthesis of gold nanoparticlesdispersed within pores of mesoporous silica induced by ultrasonic irradiation and its characterization,Mater.Res.Bull.36(2001)335–342.[31]S.M.Zhu,D.Zhang,J.J.Gu,J.Q.Xu,J.P.Dong,J.L.Li,Biotemplate fabrication ofSnO 2nanotubular materials by a sonochemical method for gas sensors,J.Nanopart.Res.12(2010)1389–1400.Fig.11.Kinetic plots based on the data of Fig.10.18(2011)1082–10901089。
A 0.8V 64×64 CMOS imager with integrated sense-and-stimulus pixel for artificial retina

A 0.8V 64x64 CMOS Imager with Integrated Sense-and-Stimulus Pixel for Artificial Retina ApplicationsChih-Lin Lee and Chih-Cheng HsiehSignal Sensing and Application LaboratoryDepartment of Electrical Engineering, National Tsing Hua UniversityHsinchu, Taiwan, R.O.CEmail: g9761571@.twAbstract —A 0.8V 64x64 CMOS Image Sensor (CIS) array with integrated sense-and-stimulus (SAS) pixel is presented in this paper. The in-pixel photon-to-biphasic-current converter (sense) and balanced current-mode stimulator (stimulus) are proposed to achieve a high integration and low power solution for high-resolution vision recovery. Two operation modes as programming (PG) mode and implanted (IP) mode are integrated in this design for multiple purposes. In PG mode, the output pattern of current stimulator array is programmable with external addresses for patterned electrical stimulus experiments of retina. In IP mode, the chip is fully functional with minimized number of I/O as 4 optimized for implant operation. A 64x64 SAS pixel array with 30x30 um2 pixel size has been designed and fabricated in TSMC 0.18um CIS technology. Measurement results show a 0.01uA/lux conversion gain and the maximum driving capability of biphasic neural stimulation current pulse is 10μA with a 10kΩ electrode model. It achieves the highest array resolution as 4096 pixels operated at 0.8V, 19.5fps, and 9.6mW.I.I NTRODUCTIONOR the blind people with age related macular degeneration (AMD) and retinitis pigmentosa (RP), blindness results from loss of photoreceptors, but other retinal neurons maintain an active connection to the brain [1]. In previous works, MOS image sensors based on epiretinal and subretinal implants to restore useful eyesight have been proposed and applied for helping blind disease. Over 1000 pixels with an area less than 5x5mm2 are needed to provide a useful vision. A retinal stimulator needs several key features in order to be useful: low power consumption, small size, long lifetime, reliability and few external components [2]. The goal is to stimulate the healthy retinal neurons for reconstructing the vision patterns using a biphasic current pulses. The pulse frequency modulation (PFM) photo sensor has many advantages such as asynchronous operation, digital output, wide dynamic range and low voltage operation [3]. A inverter-based PFM sensor with in-pixel biphasic current driver has been reported in [4]. Lower power supply voltage provides longer battery life and less heat generates which can protect the eye ball.In this work, a 64×64 pixels comparator-based PFM CIS array with in-pixel biphasic current output, which can replace the function of damaged retinal cell, has been proposed. For image sensors, the pulse-modulation-based (PM) readout circuit, such as the pulse-frequency-modulation (PFM) image sensor, converts the photo-generated charge difference of sensor node to modulated output. After that, the in-pixel biphasic current pulses generator and electrode will be implemented to achieve an on-chip photon-to-biphasic current conversion for neural stimulation application. The comparator-based PFM CIS comparison is designed to achieve a better uniformity compared to previous design of inverter-based PFM CIS [4]. Another advantage of comparator-based PFM CIS is that adjusting the reference voltage node of the comparator also can easily control the gain response of the PFM CIS. The block diagram of proposed chip and the signal conversion in the pixel are shown in Fig.1.The outline of this paper is as follow. In section II, the architecture of the pixel is described. In section III, functions of the chip’s operation mode are being described. Then, the measurement results are summarized in section IV. Finally, the conclusions are drawn in section V.II.C HIP A RCHITECTURE AND O PERATION M ODEThe proposed CIS chip provides two operation modes as programming (PG) mode for the stimulus experiment and implanted (IP) mode for the in vivo operation. The detailed descriptions are as follow:A.Programming ModeAn example with programming pattern “heart” is shown in Fig.2. In PG mode, the sensor block is disabled and the biphasic current output stage is keep enable. The pixels of arbitrary pattern are addressed by programming external signal. The H and V decoder in PG mode are used to program the pattern. It consists of shift register arrays to carry the programming data in sequentially accordance with a clock signal. The proposed PG mode provides an effective solution of patterned electrical stimulus experiments of retina. The amplitude of the biphasic current pulse is also adjustable by varying the external bias level.F IEEE Asian Solid-State Circuits ConferenceNovember 14-16, 2011 / Jeju, KoreaBlock diagram of a pixel circuit for biphasic current pulse stimulationBiphasic Current driverElectrodeOutputFig.1 Block diagram of the proposed chipFig.2 An example of programming modeB. Implantation ModeFor the applications of artificial retina, in vivo operation is inevitable. Therefore, a retina chip has to be realized with a minimum number of input/output (I/O) nodes. In IP mode, all signals such as digital control clocks, addressing, and analog biasing voltages are generated internally on chip. Thus, only four I/Os (power, ground, system clock, and biphasic-reference voltage) need to be connected to the chip. For power limitation of in vivo operation, the stimulus currents output single row at a time progressively. The total I/O numbers for different modes are summaries in Table I. With proper sharing design of interface, the proposed chip is fully functional with only 4 I/Os, that is, 4 external wires in IP mode.TABLE IPG Mode IP Mode I/O number 14 4III. SAS P IXEL A RCHITECTURE AND C IRCUITA 0.8V 64x64 CMOS Image Sensor (CIS) array with integrated sense-and-stimulus (SAS) pixel is presented. The pixel consists of two components: comparator-based PFM photo sensor and biphasic current generator which includes a frequency-to-voltage converter.A. Comparator-based PFM photosensorThe schematic of the proposed PFM photo sensor cell is shown in Fig. 3. It is composed of a photodiode, a comparator, a reset transistor Mr, and a feedback loop with a delay chain (inverter chain). The comparator converts the difference between the local luminance signal V pd and threshold terminal V th to the PFM circuit’s output. When V pd is lower than V th , the output changes the state and is feedback through a delay chain to reset the sensor node. It results in a pulse generation and the frequency is proportional to the light intensity. The delay chain is used to implement the necessary reset pulse width and maintain the oscillation condition of the loop. Since the full-well of sensor node is self-reset and re-used through the feedback loop, the dynamic range of PFM sensor is much higher than the conventional image sensor. The ultra-low voltage operation feature of the inverter-based PFM image sensor has been demonstrated in [4], where it was operated under a 0.6-V power supply voltage. But the output frequency of inverter-based PFM depends the threshold of the inverter which leads to the possibility mismatch errors. The comparator-based design generates output frequency with a better uniformity relied on amplifier’s gain instead of device’s threshold voltage, but it requires a higher operational supply as 0.8V. A tunable front-stage gain is also achieved by adjusting the voltage of V th . With this front-stage tunable gain, the dynamic range and sensor SNR can be optimized depends on applications.Biphasic current generatorAn in-pixel frequency-to-voltage (F-V) converter followed by a voltage-to-biphasic-current generator is proposed to provide the electric stimulus. As shown in Fig. 3, the F-V converter switches the current I sw and integrates the charge on the capacitor C int. An adjustable integration slope on capacitor C int is achieved by scaling the current I sw. Through the level shifter (M psf), the converted voltage is then fed to the voltage controlled current source. The generated current is then mirrored to as a current sink and a source of electrode, respectively. Optimized matching between the negative and positive current level is important to deliver a good charge balance [5]. Charge balancing is a major concern in functional electrical stimulation, since any excess charge accumulation over time leads to electrolysis with electrode dissolution and tissue destruction [6]. The biphasic pulse width, polarity, and output rate are adjustable by controlling the timing of the φ1 and φ2. After stimulation, the electrode potential value is then pullback to the reference voltage V ref by turning on the switch SW ref through the clock φ3. It removes the charge residue on the electrode after every stimulation cycle to avoid the neuron memory effect and possible physiological damage.IV.P ROTOTYPE C HIP AND M EASUREMENT R ESULTThe microphotograph of the fabricated chip (3 mm × 3 mm) in TSMC 0.18 μm CMOS image sensor (CIS) technology is shown in Fig. 4. The pixel layout is shown in Fig. 5. Each 30x30 μm2 pixel size contains a 300μm2 n-diffusion/p-substrate photodiode results in a 33.3% fill factor. An in-pixel electrode pad window is embedded for further electrode formation.Figure 6 shows the measured sensitivity of the comparator-based PFM sensor operated at 1.8V and 0.8V, respectively . The response between input light intensity (lux) and output frequency (Hz) is linear as expectation. It shows a higher lux-to-Hz gain at 0.8V due to the lower reset level and smaller signal range fed to comparator. The measured conversion gain (frequency/illumination) is 13.8 (Hz/lux) and 8 (Hz/lux) at 0.8V and 1.8V supply voltage, respectively. Fig. 7 shows the output current of the biphasic current generator with an electrode model (10kΩ) under illumination. The supply voltage determines the available swing range of the biphasic pulse. The maximum driving capability of biphasic neural stimulation currents are ±50μA and ±10μA at 1.8V and 0.8V supply (with 10kΩ loading), respectively. The reconstructed images from biphasic current output level is shown in Fig. 8.It shows that the output pattern is quite clear which potentially help restoring vision with high resolution. The image of 0.8V operation is quite noisy due to the small available signal swing. The measured results of the fabricated prototype chip are summarized in Table II. The image quality, power consumption, and driving capability of biphasic current output are all tradeoff between 1.8V and 0.8V operationFig.4 Microphotograph of the fabricated chipFig. 5 Pixel layoutBasic circuit of the SAS pixelFig. 6 Sensitivity of the PFM photosensorFig. 8 The captured image from prototype chip (a) Vdd=1.8V (b)Vdd=0.8VV. C ONCLUSIONWe are developing a retinal chip in hopes of restoring vision to the patients. The design of a 4096 pixels comparator-based PFM CMOS image sensor array with in-pixel biphasic current pulse driver has been presented. The main work is a photon-to-biphasic current conversion system on chip operated at 1.8V and 0.8V supply voltage. A tunable front-gain by adjusting the reference voltages of comparator is implemented. The resulting biphasic pulse width, polarity, and output rate are all tunable depends on applications and environments.TABLE IISpecificationsTechnology TSMC CIS 0.18-umSupply voltage 1.8V0.8VNumber of pixels64x64 Pixel size30 μm × 30 μmFill factor 33.3% Frame rate19.5fps PFM output frequency100Hz~18kHzPower consumptionPixel (without current driver)4.2uW 2.2uW Full chip @ Implantation mode>20mW >9.6mWPFM conversion gain 8 Hz/lux 13.8 Hz/luxBiphasic current(with 10k Ω loading) ±50μA±10μAA CKNOWLEDGMENTThe authors would like to thank Prof. L.S. Fan, Prof. K.T. Tang, S.F. Yeh, and M.T. Chung for supporting this work.R EFERENCES[1] L. Theogarajan, J. Wyatt, J. Rizzo, B. Drohan, M. Markova, S. Kelly, G.Swider, M. Raj, D. Shire, M. Gingerich, J. Lowenstein, B. Yomtov, “Minimally Invasive Retinal Prosthesis,” IEEE ISSCC, pp. 99-108, 2006.[2] M. Ortmanns, A. Rocke, M. Gehrke, H.J. Tiedtke, “A 232-ChannelEpiretinal Stimulator ASIC,” IEEE J. Solid-State Circuits, vol. 42, pp. 2946–2959, 2007.[3] J. Ohta, Smart CMOS Image Sensor and Applications, CRC Press,Boca Raton, FL, 2007.[4] C.L. Lee, C.C. Hsieh, “A 0.6V CMOS Image Sensor with In-PixelBiphasic Current Driver for Biomedical Application,” will be published in ISCAS , 2011.[5] A. Rothermel, L. Liu, N.P. Aryan, M. Fischer, J. Wuenschmann, S.Kibbel, and A. Harscher, “A CMOS chip with active pixel array and specific test features for subretinal implantation,” IEEE J. Solid-State Circuits, vol. 44, pp. 290–300, 2009.[6] K. Sooksood, T. Stieglitz, and M. Ortmanns, “An Active Approach forCharge Balancing in Functional Electrical Stimulation,” IEEE Trans Biomed. Circuits Syst., vol. 4, no.3, pp. 162–170, 2010.。
通信感知一体化混合波束赋形技术
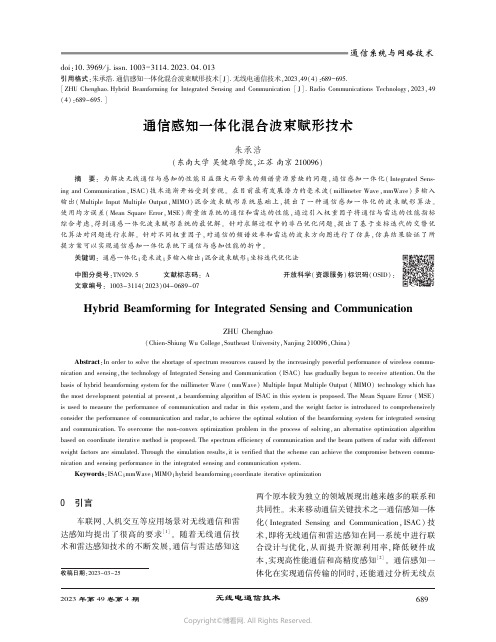
doi:10.3969/j.issn.1003-3114.2023.04.013引用格式:朱承浩.通信感知一体化混合波束赋形技术[J].无线电通信技术,2023,49(4):689-695.[ZHU Chenghao.Hybrid Beamforming for Integrated Sensing and Communication [J].Radio Communications Technology,2023,49(4):689-695.]通信感知一体化混合波束赋形技术朱承浩(东南大学吴健雄学院,江苏南京210096)摘㊀要:为解决无线通信与感知的性能日益强大而带来的频谱资源紧缺的问题,通信感知一体化(Integrated Sens-ing and Communication,ISAC)技术逐渐开始受到重视㊂在目前最有发展潜力的毫米波(millimeter Wave,mmWave)多输入输出(Multiple Input Multiple Output,MIMO)混合波束赋形系统基础上,提出了一种通信感知一体化的波束赋形算法㊂使用均方误差(Mean Square Error,MSE)衡量该系统的通信和雷达的性能,通过引入权重因子将通信与雷达的性能指标综合考虑,得到通感一体化波束赋形系统的最优解㊂针对求解过程中的非凸优化问题,提出了基于坐标迭代的交替优化算法对问题进行求解㊂针对不同权重因子,对通信的频谱效率和雷达的波束方向图进行了仿真,仿真结果验证了所提方案可以实现通信感知一体化系统下通信与感知性能的折中㊂关键词:通感一体化;毫米波;多输入输出;混合波束赋形;坐标迭代优化法中图分类号:TN929.5㊀㊀㊀文献标志码:A㊀㊀㊀开放科学(资源服务)标识码(OSID):文章编号:1003-3114(2023)04-0689-07Hybrid Beamforming for Integrated Sensing and CommunicationZHU Chenghao(Chien-Shiung Wu College,Southeast University,Nanjing 210096,China)Abstract :In order to solve the shortage of spectrum resources caused by the increasingly powerful performance of wireless commu-nication and sensing,the technology of Integrated Sensing and Communication (ISAC)has gradually begun to receive attention.On thebasis of hybrid beamforming system for the millimeter Wave (mmWave)Multiple Input Multiple Output (MIMO)technology which hasthe most development potential at present,a beamforming algorithm of ISAC in this system is proposed.The Mean Square Error (MSE)is used to measure the performance of communication and radar in this system,and the weight factor is introduced to comprehensively consider the performance of communication and radar,to achieve the optimal solution of the beamforming system for integrated sensingand communication.To overcome the non-convex optimization problem in the process of solving,an alternative optimization algorithm based on coordinate iterative method is proposed.The spectrum efficiency of communication and the beam pattern of radar with differentweight factors are simulated.Through the simulation results,it is verified that the scheme can achieve the compromise between commu-nication and sensing performance in the integrated sensing and communication system.Keywords :ISAC;mmWave;MIMO;hybrid beamforming;coordinate iterative optimization收稿日期:2023-03-250 引言车联网㊁人机交互等应用场景对无线通信和雷达感知均提出了很高的要求[1]㊂随着无线通信技术和雷达感知技术的不断发展,通信与雷达感知这两个原本较为独立的领域展现出越来越多的联系和共同性㊂未来移动通信关键技术之一通信感知一体化(Integrated Sensing and Communication,ISAC)技术,即将无线通信和雷达感知在同一系统中进行联合设计与优化,从而提升资源利用率,降低硬件成本,实现高性能通信和高精度感知[2]㊂通信感知一体化在实现通信传输的同时,还能通过分析无线点的反射㊁散射等特性,对目标信息进行定位和识别[3]㊂通信与感知的融合可以让二者实现技术共享,在满足高性能通信的同时满足复杂多样的感知需求[4]㊂该技术具有超越传统移动通信网络连接的潜力,可以开辟民用无人机㊁智慧交通等全新业务,因此受到了学界的广泛关注[5]㊂波束赋形技术是通信感知一体化的关键技术之一㊂文献[6]提出了通信感知一体化的波束赋形方案,在通信目标信噪比的约束下以目标估计误差为优化目标㊂文献[7]在相同的约束条件下使发射矩阵接近理想的雷达方向图来提高雷达感知的性能㊂为了解决射频资源紧缺的问题,5G将毫米波(millimeter Wave,mmWave)频段写入标准,用于提升传输速率㊂毫米波通信一般使用大规模多输入多输出(Multiple Input Multiple Output,MIMO)技术来增大信号强度[8]㊂随着天线阵列规模的增加,传统的全数字结构成本已经难以承担,因此毫米波通信使用将数字与模拟波束赋形结合起来的混合波束赋形技术㊂基于毫米波频段的通感一体化波束赋形技术也得到了广泛的关注㊂文献[9]提出了通信感知一体化系统的混合波束赋形方案,在满足雷达方向图的条件下,使混合波束赋形矩阵接近理想通信矩阵㊂该方案具有较低的复杂度,且雷达性能较高㊂但是在此方案下通信性能受到抑制,无法实现通信和感知性能的权衡㊂文献[10]采用正交匹配追踪算法得到最优波束赋形矩阵,该算法迭代速度较快,然而在大数据量情况下复杂度较高,且迭代过程中会产生累积误差并影响最终结果㊂现有的研究大多将优化算法的目标设计为使混合波束赋形矩阵逼近全数字波束赋形矩阵,并且通常会在约束通信或感知一者的前提下优化另一者的性能㊂这样做的缺点在于性能上会有所损失,最终求出的结果也不会是最优的㊂因此,针对毫米波MIMO下通感一体化的性能要求,本文提出了基于最小均方误差(Mean Square Error,MSE)准则设计的混合波束赋形算法㊂通过在均方误差指标中引入因子使得优化算法与信道噪声能量相关联,使设计更加准确,同时简化了求解过程㊂通过引入辅助酉矩阵使理想雷达发射矩阵与一体化下的雷达发射矩阵维度相同,可以直接进行均方误差的计算㊂优化的目标函数含有多个待优化变量,难以直接求解,因此本文提出了交替迭代优化算法㊂在假设其他优化目标为最优解的情况下单独优化一个目标,通过不断交替循环实现系统的最优解㊂在求解模拟波束赋形矩阵时,相移器阵列受恒模约束的影响,该问题是非凸优化问题㊂针对该问题,本文提出了坐标迭代优化法来求出该优化问题的最优解㊂仿真结果表明该算法较好地兼顾了通信与感知的性能,实现了二者的融合㊂1㊀毫米波通信感知一体化系统理论基础1.1㊀毫米波大规模MIMO技术5G及今后技术的发展离不开通信速率的不断提高㊂由奈奎斯特第一准则可知,通信速率与信号的带宽成正比㊂4G技术所使用的频段较低,缺乏足够的频带资源继续拓展带宽㊂因此,为了继续提高通信速率,需要利用更高频段的毫米波段㊂相比于中低频段,毫米波段拥有数十倍以上的广阔频段,可以解决带宽资源的紧张问题[11],在毫米波段下的通信与感知性能也能得到极大的提高[12]㊂然而,毫米波也有着不容忽视的缺点,根据弗里斯传输公式[13],接收功率与波长成正比,毫米波更短的波长意味着更大的传输损耗㊂为了弥补这种损耗,在应用中多采用大规模MIMO技术对其进行补偿㊂以一维均匀排布的天线阵列为例,其天线间隔应大于半波长㊂毫米波的波长极短,因此天线间隔在毫米波段下极小,可以实现大规模MIMO传输㊂1.2㊀混合波束赋形系统在传统的全数字波束赋形系统下,每根天线都必须配备一条可以任意改变信号幅度和相位的射频链路㊂然而在大规模MIMO系统中,天线的数量激增,已无法负担为每根天线加装射频链路的巨大成本[14]㊂因此,有研究者提出了使用混合波束赋形技术㊂从图1可以看出,混合波束赋形系统的特点在于使用数个相移器构成模拟波束赋形矩阵[15],减少了数字波束赋形矩阵中射频链路的数量,在很大程度上降低了建设成本㊂其中,传输信号维度为N s,使用了N RF条射频链路,发送天线数量为N t,满足关系N sɤN RF≪N t㊂图1㊀毫米波MIMO系统混合波束赋形方案Fig.1㊀Hybrid beamforming scheme formmWave MIMO system1.3㊀通信感知一体化波束赋形技术在通信感知一体化系统中,同一种波形被同时运用于通信传输和雷达感知,这二者的功能都能通过MIMO混合波束赋形系统实现㊂因此,在求解相应的波束赋形矩阵时,可以做到同时优化通信和感知的性能,这实现了通信与感知性能的兼顾与折中,与一体化的思想一致㊂2㊀基于最小MSE准则的一体化波束赋形设计2.1㊀通信模型在混合波束赋形系统中,用户接收到的信号yɪC N sˑ1可以表示为:y=W H HF RF F BB s+W H n,(1)式中:sɪC N sˑ1为发送的数据信号向量,满足关系E(ss H)=I N s,F BBɪC N RFˑN s为数字波束赋形矩阵, F RFɪC N tˑN RF为模拟波束赋形矩阵,该矩阵仅提供相位变化,因此所有元素的模为1㊂HɪC N rˑN t为信道矩阵,N r为接收端的天线数量,WɪC N rˑN s为接收端的全数字波束赋形矩阵,nɪC N rˑ1为信道噪声矢量,服从均值为0㊁方差为σ2的复高斯分布㊂对于均匀线阵,其阵列响应矢量为:a(θ)=1㊀N[1,e j kd sin(θ),e j2kd sin(θ), ,e j(N-1)kd sin(θ)]T,(2)式中:k=2πλ,d为阵元间隔,通常取d=λ/2,N为天线数,θ为到达角或离开角㊂在毫米波频段下,信道矩阵为Saleh-Valenzuela 模型[16],可以表示为:H=㊀NtN rLðL l=1αl a r(θr,l)a H t(θt,l),(3)式中:L为多径数,αl为第l条传输路径的信道增益,服从标准复高斯分布,θr,l为第l条传输路径的到达角,θt,l为第l条传输路径的离开角㊂2.2㊀感知模型MIMO的雷达发射波束方向图为[17]:P(θ)=a H t(θ)R s a t(θ),(4)式中:R sɪC N tˑN t为发射信号的协方差矩阵,可以表示为:Rs=E(F RF F BB ss H F H BB F H RF)=F RF F BB E(ss H)F H BB F H RF=F RF F BB F H BB F H RF㊂(5)假设雷达感知的目标数量为K,相对于基站的离开角为{θt,1,θt,2, ,θt,K}㊂由式(3)可知,信道矩阵表示为L个不同离开角和到达角的散射路径的求和㊂信道的前K个散射路径即为雷达感知K个目标的路径㊂因此信道前K个路径的离开角应为雷达感知的离开角,即为{θt,1,θt,2, ,θt,K},剩下的L-K个离开角和L个到达角均服从[-π/2,π/2]的均匀分布㊂2.3㊀通信感知一体化的最小MSE模型在一体化系统的设计过程中,衡量通信系统性能的主要标准为误比特率(Bit Error Ratio,BER)等㊂在传统波束赋形设计中,通常通过降低MSE来达到降低误比特率的目的㊂本文将这一指标运用到一体化混合波束赋形的应用范围内,目的也是通过降低通信和雷达感知的均方误差来优化通信和感知的各项性能㊂通信性能的MSE定义为接收信号与原始信号的均方误差:MSE c=E( β-1y-s 2F)=E( β-1(W H HF RF F BB s+W H n)-s 2F)= tr(β-2W H HF RF F BB F H BB F H RF H H W-β-1W H HF RF F BB-β-1F H BB F H RF H H W+σ2β-2W H W+I Ns)(6)式中:引入的β因子可以将之后在功率约束下的优化求解问题大大简化,变成以β为优化目标的子问题㊂由雷达感知的波束图公式可知,雷达的波束设计等价于设计雷达的协方差矩阵㊂理想的全数字雷达发射矩阵F radɪC N tˑK为:F rad =[a t (θt ,1),a t (θt ,2), ,a t (θt ,K )]㊂(7)然而,混合波束赋形系统中的雷达发射矩阵为F RF F BB ɪCN t ˑN s,与理想的发射矩阵维度不一致,因此二者不能直接进行MSE 的计算㊂为使二者维度一致,可以引入一个辅助酉矩阵F u ɪC K ˑN s,其满足关系F u F H u =I K ,这样,理想雷达的发射矩阵可以表示为F r =F rad F u ɪCN t ˑN s㊂可以看到,引入辅助酉矩阵后,理想雷达的发射矩阵与混合波束赋形中的发射矩阵维度一致,并且原来理想雷达的方向没有改变,维持了原始的性能㊂辅助酉矩阵可以通过以下的优化问题解出:min F uF c -F rad F u 2Fs.t.㊀F u F Hu=I K{,(8)式中:F c 为理想的通信全数字波束赋形矩阵㊂对信道矩阵进行奇异值分解:H =U V H ㊂(9)取V 的前N s 列即为通信全数字波束赋形矩阵F c ㊂该优化问题表明构造辅助酉矩阵应尽可能减小全数字波束赋形下通信与雷达感知的差异,提高一体化的性能㊂该问题类似于正交普鲁克问题,可以求得F u的闭式解为[18]:F u =U 1CV H 1,(10)式中:U 1和V 1来自于F H rad F c 的奇异值分解F Hrad F c=U 11V H 1,C =[I K ,O K ˑ(N s -K )]㊂由此,雷达感知的MSE 可以定义为:MSE r = F RF F BB -F r 2F =tr(F RF F BB F H BB F H RF -F RF F BB F H r -F r F H BB F H RF +F r F Hr )㊂(11)在一体化的混合波束赋形设计中,需要同时以通信和雷达的性能作为优化对象,因此优化问题的目标函数应同时包含二者的均方误差㊂通感一体化下的混合波束赋形优化问题可以表示为:min W ,F RF ,F BB ,βρMSE c +(1-ρ)MSE r s.t.㊀(F RF )ij =1,∀i ,j F RF F BB 2FɤP ìîíïïïï,(12)式中:ρɪ[0,1]为一权重因子,代表通信性能在优化中所占的比重㊂该优化问题需要考虑模拟波束赋形矩阵的恒模约束和混合波束赋形矩阵的功率约束㊂3㊀基于交替迭代优化算法求解波束赋形设计3.1㊀基于坐标迭代的交替优化上文中通感一体化下的混合波束赋形优化问题涉及到4个待优化变量,难以直接求解㊂因此,可以每次在固定其他变量的条件下交替优化一个变量,通过多轮这样的迭代优化使目标函数最终落入目标区间内㊂①关于W 的子问题可以表示为:min W MSE c ㊂(13)将目标函数MSE c 对W 求偏导并使结果等于零可以得到W 的闭式解为:W =(HF RF F BB F H BB F H RF H H +σ2β-2I N r)-1ˑβ-1HF RF F BB ㊂(14)②关于β的子问题,由于存在发射功率的限制,只有在发射功率达到最大时β才能达到最优值㊂令F bb =β-1F BB 以简化表达,可以得到在发射功率最大时的β值为:β=P -12(tr(F RF F bb F HbbF H RF))-12㊂(15)从求解过程可以看出,若按照未引入β因子的传统MSE 标准来优化,则需要引入拉格朗日乘子将功率约束条件利用起来再进行复杂的求解,但在引入β因子后,就可以将功率约束分解为β的子问题求得闭式解,这无疑大大简化了算法流程㊂③关于F BB 的子问题可以表示为:min F BBρMSE c +(1-ρ)MSE r ㊂(16)将目标函数对F BB 求偏导并使结果等于零可以得到F BB 的闭式解为:F BB =(ρβ-2F H RF H H WW H HF RF +(1-ρ)F HRF F RF )-1ˑ(ρβ-1F H RF H H W +(1-ρ)F HRF F r )㊂(17)④关于F RF 的子问题可以表示为:min FRFρMSE c +(1-ρ)MSE rs.t.㊀(F RF )ij =1,∀i ,j{㊂(18)约束条件(F RF )ij =1,∀i ,j 使得上述优化问题是非凸的,这使得问题的理论求解十分困难㊂本文针对该问题提出坐标迭代优化法对其进行求解㊂F RF 的优化问题可以表示为:J (F RF )=ρMSE c +(1-ρ)MSE r =ρtr(β-2W H HF RF F BB F H BB F H RF H H W -β-1W HHF RF F BB -β-1F H BB F H RF H H W +σ2β-2W H W +I N s)+(1-ρ)tr(F RF F BB F H BB F H RF -F RF F BB F H r -F r F H BB F HRF +F r F H r )=ρtr(A l )+(1-ρ)tr(B l )+ρtr(β-2W H HV RF V BB V H BB V H RF H H W -2β-1W H HV RF V BB )+(1-ρ)tr(V RF V BB V H BB V H RF -2V RF V BB F H r ),(19)式中:A l =β-2W H HF -l RF F -l BB (F -l BB )H (F -l RF )H H H W -β-1W H HF -l RF F -l BB-β-1(F -l BB )H (F -l RF )H H H W +σ2β-2W H W +I Ns,(20)B l =F -l RF F -l BB (F -l BB )H (F -l RF )H -F -l RF F -l BB F Hr -F r (F -l BB )H (F -l RF )H +F r F H r,(21)式中:F -l RF 为矩阵F RF 移除第l 列后的子矩阵,F -l BB 为矩阵F BB 移除第l 行后的子矩阵,V RF 为矩阵F RF第l 列的矢量,V BB 为矩阵F BB 第l 行的矢量㊂固定矩阵F RF 其他列不变,将第l 列的矢量V RF单独作为变量优化,原优化问题可以转化为:min F RFρtr(β-2W H HV RF V BB V H BB V H RF H H W -2β-1W HHV RF V BB )+(1-ρ)tr(V RF V BB V HBBV H RF-2V RF V BB F H r)s.t.㊀(V RF )n =1,∀n ㊂(22)该优化问题同样可以用类似方法处理,每次固定V RF ,其他元素不变,将第n 个元素V RF (n )作为变量求最优解㊂令H w =W H H ,F v =V BB F H r ,由于模拟波束赋形矩阵仅有相移的功能,可令V RF (n )=e j θn ,则目标函数中与V RF (n )有关的项为:J (θn )=ρðN sm =1[β-2H w (m ,n )V BB (m )2ej2θn-2β-1H w (m ,n )ˑV BB (m )e j θn]+(1-ρ)ðN s m =1V BB (m )2e j2θn-2(1-ρ)F v (n )e j θn ㊂(23)令:X n =ðN sm =1H w (m ,n )V BB (m )2,(24)Y n =ðN s m =1H w (m ,n )V BB (m )㊂(25)求J (θn )关于θn 的偏导,使其等于零,可以求得V RF (n )的最优解为:V RF (n )=ej θn=ρβ-1Y n +(1-ρ)F v (n )ρβ-2X n +(1-ρ) V BB 2F㊂(26)对F RF 中的每个元素依次使用上述算法,即可求得当前条件下F RF 的最优解㊂基于坐标迭代的交替优化算法的详细步骤如算法1所示㊂算法1㊀交替优化算法输入:输入:H ,N s ,N RF ,N t ,N r ,P ,σ2,ρ,I max ,S min输出:F BB ,F RF ,W ,β1.㊀在约束条件(F RF )ij =1,∀i ,j 下随机初始化矩阵F RF2.根据式(9)得到通信全数字波束赋形矩阵F c ,初始化F BB =F -1RF F c3.初始化β=P -1/2(tr(F RF F BB F H BB F H RF ))-1/24.for i =1,2, ,I max do5.㊀㊀根据式(15)更新β6.㊀㊀根据式(14)更新W7.㊀㊀根据式(26)用坐标迭代优化法更新F RF 8.㊀㊀根据式(17)更新F BB9.㊀㊀根据式(6)和式(11)计算MSE c 和MSE r10.㊀㊀if ρMSE c +(1-ρ)MSE r <S min then11.㊀㊀㊀结束循环12.㊀㊀end if13.end for3.2㊀仿真分析本节通过仿真结果来分析使用基于坐标迭代的交替优化算法求解的一体化混合波束赋形系统的性能㊂仿真中,发射天线数N t =64,接收天线数N r =8,N RF =N s =4,将每条射频链路使用的发射功率归一化为1,则总系统的归一化发射功率P =4,毫米波信道多径数L =10[19],雷达检测目标K =3,离开角分别为[-45ʎ,0ʎ,45ʎ],信道中其余离开角和到达角均服从[-π/2,π/2]的均匀分布㊂图2为不同权重因子ρ下频谱效率随信噪比变化的曲线㊂可以看出,随着通信性能权重ρ的增大,混合波束赋形的频谱效率也在增大,且越来越接近全数字波束赋形下的频谱效率㊂当ρ=1时,混合波束赋形系统只考虑通信的性能,此时的频谱效率与全数字状态非常接近㊂因此可以看出,权重因子ρ的大小在优化过程中会影响一体化系统的通信性能㊂图2㊀不同权重下频谱效率随信噪比的变化曲线Fig.2㊀Curve of spectral efficiency versus signal-to-noise ratio with different weights图3为不同权重下雷达波束图与理想全数字雷达波束图的比较㊂由于ρ值越小代表雷达性能在优化中占比越大,可以看到,随着ρ值的不断下降,一体化系统下的雷达波束图与全数字下的波束图越来越接近㊂在ρ=0.7时,雷达波束存在较大的旁瓣,这会较大地干扰正确的检测目标;ρ=0.5时,旁瓣干扰仍然存在,但此时主瓣强度明显高于旁瓣,可以进行有效的检测;ρ=0.3时,旁瓣强度被显著抑制,这时的旁瓣干扰很小,主瓣方向的波束容易分辨,雷达感知的精度较高,能够准确地识别目标方位㊂由上述分析可知,本文提出的基于坐标迭代的交替优化算法在保障通信性能的同时可以实现较高的雷达感知精度,且可以通过改变权重ρ值灵活地调整通信与感知性能的占比,实现二者的权衡,达到通感一体化的效果㊂图3㊀不同权重时的雷达波束方向图Fig.3㊀Radar beam patterns with different weight factors4 结论本文使用了毫米波信道下的混合波束赋形技术实现通信感知一体化㊂通过引入因子β导出基于最小均方误差准则的通信性能优化问题,并引入辅助酉矩阵,让理想雷达发射矩阵与混合波束赋形矩阵保持维度相同,得到了基于雷达感知性能的优化问题㊂接着利用权重因子ρ结合两方面性能提出了通感一体化下的混合波束赋形优化问题㊂针对非凸优化问题提出了基于坐标迭代的交替优化算法,完成了对波束赋形优化问题的求解㊂仿真结果表明,该算法能够很好地实现通信与感知性能的折中,即在不同权重下通信与感知的性能都能有所保证,实现了通信感知一体化的效果㊂参考文献[1]㊀LIU F,CUI Y,MASOUROS C,et al.Integrated Sensingand Communications:Towards Dual-functional WirelessNetworks for 6G and Beyond[J].IEEE Journal on Select-ed Areas in Communications,2022,40(6):1728-1767.[2]㊀吴晓文,焦侦丰,刘冰,等.面向6G 的卫星通感一体化[J].移动通信,2022,46(10):2-11.[3]㊀LIU Y J,LIAO G S,XU J W,et al.Adaptive OFDM Inte-grated Radar and Communications Waveform Design Based on Information Theory [J].IEEE communications letters,2017,21(10):2174-2177.[4]㊀高飞,王文剑,刘建国,等.通感一体化融合的研究及其挑战[J].移动通信,2022,46(5):45-51.[5]㊀李萍,郭晓江.通感一体化关键技术与应用[J].中兴通讯技术,2023,29(2):72-78.[6]㊀LIU F,MASOUROS C.Joint Beamforming Design for Ex-tended Target Estimation and Multiuser Communication [C]ʊ2020IEEE Radar Conference (RadarConf20).Florence:IEEE,2020:1-6.[7]㊀LIU X,HUANG T,SHLEZINGER N,et al.Joint TransmitBeamforming for Multiuser MIMO Communications and MIMO Radar[J].IEEE Transactions on Signal Process-ing,2020,68:3929-3944.[8]㊀VOOK F W,THOMAS T A,VISOTSKY E.Massive MIMOfor mmWave Systems [C]ʊ201448th Asilomar Confer-ence on Signals,Systems and Computers.Pacific Grove:IEEE,2014:820-824.[9]㊀LIU F,MASOUROS C,PETROPULU A P,et al.Joint Ra-dar and Communication Design:Applications,State-of-the-art,and the Road Ahead[J].IEEE Transactions on Com-munications,2020,68(6):3834-3862. [10]EL AYACH O,RAJAGOPAL S,ABU-SURRA S,et al.Spatially Sparse Precoding in Millimeter Wave MIMO Sys-tems[J].IEEE Transactions on Wireless Communica-tions,2014,13(3):1499-1513.[11]RAPPAPORT T S,SHU S,MAYZUS R,et limeterWave Mobile Communications for5G Cellular:It WillWork[J].IEEE Access,2013,1(1):335-349. [12]RAI P K,IDSØE H,YAKKATI R R,et al.Localizationand Activity Classification of Unmanned Aerial VehicleUsing mmWave FMCW Radars[J].IEEE Sensors Jour-nal,2021,21(14):16043-16053.[13]罗涛,王昊.车辆无线通信网络及其应用[J].中兴通讯技术,2011,17(3):1-7.[14]邓瑾.大规模MIMO系统中的波束空间信道估计以及混合波束成形研究[D].成都:电子科技大学,2022. [15]YU X,SHEN J C,ZHANG J,et al.Alternating Minimiza-tion Algorithms for Hybrid Precoding in Millimeter WaveMIMO Systems[J].IEEE Journal of Selected Topics inSignal Processing,2016,10(3):485-500.[16]AKDENIZ M R,LIU Y,SAMIMI M K,et limeterWave Channel Modeling and Cellular Capacity Evaluation[J].IEEE Journal on Selected Areas in Communications,2014,32(6):1164-1179.[17]CHENG Z,LIAO B,HE Z,et al.Joint Design of theTransmit and Receive Beamforming in MIMO Radar Sys-tems[J].IEEE Transactions on Vehicular Technology,2019,68(8):7919-7930.[18]VIKLANDS T.Algorithms for the Weighted OrthogonalProcrustes Problem and Other Least Squares Problems[D].Sweden:UmeåUniversitet,2006.[19]LIU F,MASOUROS C.Hybrid Beamforming with Sub-arrayed MIMO Radar:Enabling Joint Sensing and Commu-nication at mmWave Band[C]ʊICASSP2019-2019IEEE International Conference on Acoustics,Speech andSignal Processing(ICASSP).Brighton:IEEE,2019:7770-7774.作者简介:㊀㊀朱承浩㊀东南大学本科在读㊂主要研究方向:通信感知一体化㊂。
基于深度多级小波U-Net的车牌雾图去雾算法

第49卷第6期2022年6月Vol.49,No.6Jun.2022湖南大学学报(自然科学版)Journal of Hunan University(Natural Sciences)基于深度多级小波U-Net的车牌雾图去雾算法陈炳权†,朱熙,汪政阳,梁寅聪(吉首大学信息科学与工程学院,湖南吉首416000)摘要:为了解决雾天拍摄的车牌图像边缘模糊、色彩失真的问题,提出了端到端的基于深度多级小波U-Net的车牌雾图去雾算法.以MWCNN为去雾网络的主体框架,利用“SOS”增强策略和编解码器之间的跨层连接整合小波域中的特征信息,采用离散小波变换的像素-通道联合注意力块降低去雾车牌图像中的雾度残留.此外,利用跨尺度聚合增强块补充小波域图像中缺失的空间域图像信息,进一步提高了去雾车牌图像质量.仿真实验表明,该网络在结构相似度和峰值信噪比上具有明显的优势,在处理合成车牌雾图和实际拍摄的车牌雾图上,去雾效果表现良好.关键词:车牌雾图去雾;MWCNN;“SOS”增强策略;跨层连接;注意力;跨尺度聚合中图分类号:TP391.41文献标志码:ADehazing Algorithm of License Plate Fog Image Basedon Deep Multi-level Wavelet U-NetCHEN Bingquan†,ZHU Xi,WANG Zhengyang,LIANG Yincong(College of Information Science and Engineering,Jishou University,Jishou416000,China)Abstract:To solve the problem of edge blurring and color distortion of license plate images taken in foggy weather,an end-to-end depth multilevel wavelet U-Net based algorithm for license plate fog image removal is pre⁃sented.Taking MWCNN as the main frame work of the defogging network,the feature information in the wavelet do⁃main is integrated using the“SOS”enhancement strategy and the cross-layer connection between the codec.The pixel-channel joint attention block of the discrete wavelet transform is used to reduce the fog residue in the defrosted license plate image.In addition,the cross-scale aggregation enhancement blocks are used to supplement the missing spatial domain image information in the wavelet domain image,which further improves the quality of the defogging li⁃cense plate image.The simulation results show that the network has obvious advantages in structural similarity and peak signal-to-noise ratio,and it performs well in dealing with the composite plate fog image and the actual photo⁃graphed plate fog image.Key words:license plate fog image defogging;MWCNN;“SOS”enhancement strategy;cross-layer connection;attention mechanism;cross-scale aggregation∗收稿日期:2021-11-01基金项目:国家自然科学基金资助项目(No.62141601),National Natural Science Foundation of China(No.62141601);湖南省教育厅重点资助项目(21A0326),The SRF of Hunan Provincial Education Department(No.21A0326)作者简介:陈炳权(1972-),男,湖南常德人,吉首大学副教授,硕士生导师,博士†通信联系人,E-mail:****************文章编号:1674-2974(2022)06-0124-11DOI:10.16339/ki.hdxbzkb.2022293第6期陈炳权等:基于深度多级小波U-Net的车牌雾图去雾算法在大雾天气下使用光学成像器件(如相机、监控摄像头等)对目标场景或物体进行拍摄时,往往会使得图像对比度低,边缘、字符等细节信息模糊.图像去雾是图像处理中典型的不适定问题,旨在从雾天图像中复原出相应的无雾图像,作为一种提升图像质量的方法,已被广泛应用于图像分类、识别、交通监控等领域.近年来,针对不同场景(室内家居场景、室外自然场景、交通道路场景、夜间雾霾场景等)下均匀雾度或非均匀雾度图像的去雾技术引起了广泛关注与研究,但由于实际雾霾对图像影响的复杂多变性,从真实的雾天图像中复原无雾图像仍具有诸多挑战性.图像去雾技术发展至今,主要分为以下三类:基于数学模型的去雾技术,如直方图均衡[1]、小波变换[2]、色彩恒常性理论[3](Retinex)等;基于大气散射模型(ASM)和相关统计先验的去雾技术,如暗通道先验[4-5](DCP)、色衰减先验[6](CAP)、非局部先验[7](NLP)等;基于深度学习的去雾技术,如Deha⁃zeNet[8]、DCPDN[9]、AODNet[10]等.近年来,深度卷积神经网络在计算机视觉中应用广泛,2019年Liu 等[11]认为传统的卷积神经网络(CNN)在采用池化或空洞滤波器来增大感受野时势必会造成信息的丢失或网格效应,于是将多级小波变换嵌入到CNN中,在感受野大小和计算效率之间取得了良好的折中,因而首次提出了多级小波卷积神经网络(MWCNN)模型,并证实了其在诸如图像去噪、单图像超分辨率、图像分类等任务中的有效性.同年,Yang等[12]也认为离散小波变换及其逆变化可以很好地替代U-Net 中的下采样和上采样操作,因而提出了一种用于单幅图像去雾的小波U-Net网络,该网络与MWCNN结构非常相似.2020年,Yang等[13]将多级小波与通道注意力相结合设计了一种小波通道注意力模块,据此构建了单幅图像去雨网络模型.同年,Peng等[14]则将残差块与MWCNN相结合提出了一种用于图像去噪的多级小波残差网络(MWRN).2021年,陈书贞等[15]在已有的MWCNN结构上加入多尺度稠密块以提取图像的多尺度信息,并在空间域对重构图像进行进一步细化处理,以弥补小波域和空间域对图像信息表示存在的差异性,从而实现了图像的盲去模糊.为了解决大雾天气下车牌图像对比度低和边缘、字符等信息模糊不清的问题,很多研究人员开始将已有的图像去雾技术应用于车牌识别的预处理中.但大多数只是对已有图像去雾算法进行了简单改进,如对Retinex或DCP等去雾算法进行改进,直接应用于车牌检测识别中.虽然取得了一定的去雾效果,但其并没有很好地复原出车牌图像的特征,且很难应对中等雾和浓雾下的车牌图像.2020年王巧月等[16]则有意识地针对车牌图像的颜色和字符信息进行车牌图像的去雾,提高了车牌图像的质量.受上述研究的启发,本文提出一种基于深度多级小波U-Net的车牌雾图去雾算法,以端到端的方式来实现不同雾度下不同车牌类型的去雾.首先,提出了一种结合DWT、通道注意力(CA)和像素注意力(PA)的三分支结构,该结构可以对编码器每层输出特征的通道和像素进行加权处理,从而让去雾网络聚焦于车牌雾图中的有雾区域;其次,在解码器中引入“SOS”增强模块(“SOS”Block)来对解码器和下层输入的特征进行进一步融合和增强,提高去雾图像的峰值信噪比,并在U-Net编解码结构之间进行了层与层之间的连接,以此来充分利用不同网络层和尺度上的特征信息;最后,为弥补小波域和空间域之间网络表达图像信息的差异,提出了一种结合跨尺度聚合(CSA)的多尺度残差增强模块(CSAE Block),在空间域上进一步丰富网络对于图像细节信息的表达,有效地提高去雾图像的质量.1去雾网络结构本文去雾网络结构如图1所示.该网络主要分为A与B这2大模块,前者在小波域中实现对车牌雾图x LPHaze的去雾,后者在空间域上对模块A输出的无雾图像进行进一步优化,模块A 的网络结构参数见表1.整个网络结构的输出为:y LPDhaze=y B(y A(x LPHaze;θA);θB)(1)式中:y B(⋅)和y A(⋅)分别为模块A和B的输出,θA 和θB分别表示模块A和B的可学习参数.1.1小波U-Net二维离散小波变换(2D-DWT)可以实现对给定的图像I的小波域分解,分解过程可视为将图像I与4个滤波器进行卷积,即1个低通滤波器f LL和3个高通滤波器(f LH、f HL和f HH),这4个滤波器(即卷积核)分别由低通滤波器f L和高通滤波器f H构成.以Haar小波为例,该卷积核表示为:f L=12[]1,1T,f H=12[]1,-1Tf LL=LL T,f HL=HL T,f LH=LH T,f HH=HH T(2)125湖南大学学报(自然科学版)2022年图1去雾网络结构Fig.1Defogging network structure 表1模块A 网络结构参数Tab.1Network structure parameters of module A网络层层1层2层3层4类型注意力块卷积层残差组注意力块(下、上采样)卷积层残差组注意力块(下、上采样)卷积层残差组注意力块(下、上采样)卷积层残差组卷积层编码器(卷积核大小f ×f ,输出通道c )éëêêùûúú()2×2,1()1×1,4and ()1×1,16(3×3,16)éëêêùûúú()3×3,16()3×3,16×3éëêêùûúú()2×2,1()1×1,64(3×3,64)éëêêùûúú()3×3,64()3×3,64×3éëêêùûúú()2×2,1()1×1,256(3×3,256)éëêêùûúú()3×3,256()3×3,256×3éëêêùûúú()2×2,1()1×1,1024(3×3,1024)éëêêùûúú()3×3,1024()3×3,1024×3(3×3,1024)输出大小(宽W ,高H )(64,32)(64,32)(64,32)(32,16)(32,16)(32,16)(16,8)(16,8)(16,8)(8,4)(8,4)(8,4)(8,4)解码器(卷积核大小f ×f ,输出通道c )—éëêêùûúú()3×3,16()3×3,12éëêêùûúú()3×3,16()3×3,16×3—(3×3,64)éëêêùûúú()3×3,64()3×3,64×3—(3×3,256)éëêêùûúú()3×3,256()3×3,256×3————输出大小(宽W ,高H )—(64,32)(64,32)—(32,16)(32,16)—(16,8)(16,8)————层2层4层1层3层1层2层3层4离散小波变换DWT 卷积层ConvLayer 注意力块Attention Block “SOS ”增强块“SOS ”Block 残差组ResGroup 离散小波逆变换IDWT 跨尺度聚合增强块CSAE BlockTanh 层模块B模块A 层间多尺度聚合126第6期陈炳权等:基于深度多级小波U-Net 的车牌雾图去雾算法因此,2D-DWT 可以通过将输入图像I 与4个滤波器进行卷积和下采样来实现,从而获得4个子带图像I LL 、I LH 、I HL 和I HH .其操作定义如下:I LL =()f LL ∗I ↓2I LH =()f LH ∗I ↓2I H L=()f HL∗I ↓2I HH=()f HH∗I ↓2(3)其中:∗表示卷积操作;↓2表示尺度因子为2的标准下采样操作.低通滤波器用于捕获图像中光滑的平面和纹理信息,其它3个高通滤波器则提取图像中存在的水平、垂直和对角线方向上的边缘信息.同时,由于2D-DWT 的双正交性质,可以通过二维逆DWT 的精确重建出原始图像.2D-DWT 及其逆变换的分解和重建示意图如图2所示.本文将2D-DWT 及其逆变换嵌入到U-Net 网络结构中,改善原始U-Net 网络的结构.首先,对输入的3通道车牌雾图进行离散小波变换处理,输出图像的通道数变为原来的4倍,图像大小变为原来的12;然后,使用一个单独的卷积层(“3×3卷积+Lea⁃kyReLU ”)将输入图像扩展为16通道的图像;最后,在U-Net 的每层中迭代使用卷积层和离散小波变换用于提取多尺度边缘特征.1.2基于2D-DWT 的通道-像素联合注意力块(DCPA Block )在去雾网络中平等对待不同的通道和像素特征,对于处理非均匀雾度图像是不利的.为了能灵活处理不同类型的特征信息,Qin 等[17]和Wu 等[18]均采用CA 和PA ,前者主要用于对不同通道特征进行加权,而后者则是对具有不同雾度的图像像素进行加权,从而使网络更关注雾度浓厚的像素和高频区域.引入Hao-Hsiang Yang 等[13]的小波通道注意力块,本文提出了一种基于二维DWT 的通道-像素联合注意力模块,将DWT 、CA 和PA 构建并行的三分支结构,如图3所示.2D-DWT卷积3×3平均池化卷积Leaky ReluLeaky Relu 输入逐元素相乘残差组输出逐元素相加Leaky Relu 1×1卷积2×2Sigmoid 激活函数注意力块图3基于二维DWT 的特征融合注意力模块结构Fig.3Attention module structure of feature fusion basedon two-dimensional DWT其中,两分支结构的注意力块结合了PA (上分支)和CA (下分支)的特点,将具有通道数为C ,高和宽分别为H 、W 的特征图x ∈R C ×H ×W 分别输入到CA 和PA 中,前者通过平均池化将C×H×W 的空间信息转换为C×1×1的通道权重信息,而后者则通过卷积来将C×H×W 的图像转换成1×H×W.CA 由一个平均池化层、一个卷积层和一个LeakyReLU 层构成,其输出为:y CA =LeakyReLU 0.2(Conv 11(AvgPool (x)))(4)式中:y CA ∈R 1×H ×W ;Conv j i (⋅)表示卷积核大小为i ×i 、步长为j 的卷积操作;LeakyReLU 0.2(⋅)表示参数为0.2的LeakyReLU 激活函数;AvgPool (⋅)表示平均池化操作.类似地,PA 有一个卷积层和一个LeakyReLU层,但没有平均池化层,其输出为:y PA =LeakyReLU 0.2(Conv 22(x))(5)式中:y PA ∈R C ×1×1.CA 和PA 通过逐像素相加,并共用一个Sigmoid 激活函数来分别为输入图像通道和像素配置相应的权重参数,其输出为:y A =Sigmoid (y PA ⊕y CA)(6)式中:y A ∈R C ×H ×W ;⊕表示逐元素相加;Sigmoid (⋅)表示Sigmoid 激活函数.最后,和经离散小波变换、卷列行LLLI LII LH I HL HH22222222列I HL I LL I HH222HL H I H2L行HII LH 图22D-DWT 及其逆变换Fig.22D-DWT and its inverse transformation127湖南大学学报(自然科学版)2022年积层和残差组处理后的特征图进行逐元素相乘,从而实现对特征图的加权,其最终输出为:y DCPA =ResGroup (LeakyReLU 0.2(Conv 13(DWT (x))))⊗yA(7)式中:y DCPA ∈R C ×H ×W ;DWT (⋅)为离散小波变换;⊗表示逐元素相乘;ResGroup (⋅)表示残差组函数. 1.3层间多尺度聚合(LMSA )受到Park 等[19]在图像去雾任务中采用多级连接来复原图像细节信息的启示,将U-Net 编码器中前3层中DCPA Block 的输出进行跨层和跨尺度的特征聚合,使网络能充分利用图像潜在的特征信息,其结构如图4所示.假设编码器的第l 层输出的聚合特征为y lconcat ,输入解码器的第l 层特征为D l in ,其中l =1,2,3,则y lconcat =Cat((Cat i =13F l i (y l DCPA )),F up (D l +1out ))(8)D l in =LeakyReLU 0.2(Conv 11(LeakyReLU 0.2(y l SEBlock (y l concat))))(9)式中:Cat (⋅)表示级联操作;F l i (⋅)表示从第i 层到第l 层的采样操作;F up (⋅)表示上采样操作;D i +1out为解码器的第i +1层的输出.将每层聚合后的特征图x l concat 输入到SEBlock 中,自适应地调节各个通道特征,其输出为:y lSEBlock (x l concat )=Sigmoid (FC (ReLu (FC (AvgPool (xlconcat)))))(10)式中:FC (⋅)表示全连接层函数;ReLU (⋅)为ReLU 非线性激活函数.SEBlock 的结构如图5所示.平均池化全连接层全连接层ReLuSigmoid 激活函数S图5SEBlock 结构Fig.5SEBlock structure通过“LeakyRelu-Conv-LeakyRelu ”操作减少每层输出特征的通道数,输入到U-Net 解码器中前3层的“SOS ”Block 中,提升重构图像的峰值信噪比.U-Net 网络中的第4层则聚合前2层的DCPABlock 输出特征和第3层的DWT 输出特征,同样经过SEBlock 和“LeakyRelu-Conv-LeakyRelu ”操作后作为第4层的输入特征进行后续操作.其数学表达式为:y 4concat =Cat((Cat i =12F 4i (y 4DCPA )),E 3out )(11)D 4in =LeakyReLU 0.2(Conv 11(LeakyReLU 0.2(y 4SEBlock (y 4concat))))(12)其中,E 3out 表示解码器第3层的输出.1.4“SOS ”增强模块(“SOS ”Block )从Romano 等[20]和Dong 等[21]对“Strengthen-Operate-Subtract ”增强策略(“SOS ”)的开发和利用中可知,该增强算法能对输入的图像特征进行细化处理,可以提高输出图像的峰值信噪比.因此,在本文的车牌图像去雾网络解码器结构中,直接将Dong 等[21]采用的“SOS ”增强算法嵌入到U-Net 结构中,提升车牌去雾图像的质量.Dong 等[21]所采用的“SOS ”增强算法的近似数学表达式如下:J n +1=g (I +J n )-J n(13)层1DCPA Block输出层2DCPA Block输出层3DCPA Block输出编码器CCC层1“SOS ”Block层2“SOS ”Block层3“SOS ”Block解码器LeakyReLU-1×1Conv-LeakyReLU SEBlocky 2CSACaty 1coocat y 3CSACat y 2CSAEBlocky 1CSAEBlock y 3CSAEBlockD 2inD 1inD 3in图4层间多尺度聚合结构Fig.4Multi-scale aggregation structure128第6期陈炳权等:基于深度多级小波U-Net 的车牌雾图去雾算法其中:I 为输入雾图像;J n 为第n 层估计的去雾图像;I +J n 表示使用输入雾图进行增强的图像;g (⋅)表示去雾方法或者优化方法.在U-Net 中编解码器之间的嵌入方式如下:将U-Net 编解码器之间的连接方式改为了逐元素相加,即编码器第i 层输出的聚合特征D i in 加上对应的解码器的第i 层输入特征D i +1out(经上采样操作得到与D i in 相同的图片大小);将逐元素相加后的输出接入到优化单元(即残差组)中进行进一步的特征细化,随后减去解码器的第i 层输入D i +1out .通过上述嵌入方式,模块输出为:y i sos =g i (D i in +(D i +1out )↑2)-(D i +1out )↑2(14)其中:↑2表示尺度因子为2的上采样操作.其与U-Net 相结合的结构示意图如图6所示.解码器的第i +1层输出编码器的第i 层输出逐元素相减优化单元g (·)逐元素相加2D DWT2D IDWT图6“SOS ”深度增强模块Fig.6"SOS"depth enhancement module1.5跨尺度聚合增强模块(CSAE Block )为了弥补小波域中去雾网络所忽略的精细空间域图像特征信息,本文提出了一种基于残差组的跨尺度聚合增强模块(CSAE Block ),对小波域网络(模块A )最后输出的重构图像进行空间域上的图像细节特征补充.CSAE Block 结构如图7所示.CSAE Block 主要由卷积层(即“3×3卷积-Lea⁃kyReLU ”)、残差组、平均池化、CSA 和级联操作构成.首先,卷积层和残差组负责对模块A 输出的空间域图像y 模块A 进行特征提取,平均池化将输入特征分解为4个不同尺度(S 1=14,S 2=18,S 3=116和S 4=132)的输出,即:y S 1,y S 2,y S 3,y S 4=AvgPool (ResGroup (Conv 13(y 模块A)))(15)然后,CSA 实现对输入的不同尺度、空间分辨率的特征信息进行聚合,从而达到在所有尺度级别上有用信息的融合,并在每个分辨率级别上生成精细特征;最后,通过“LeakyRelu-Conv-LeakyRelu ”操作来对输入特征的通道数进行调整.该模块可以通过聚合不同尺度之间、不同分辨率之间的特征来使去雾网络获得较强的上下文信息处理能力.该聚合操作可表示如下:y SjCSA =⊕S i∈{}1,2,3,4F S j S i(y Si)(16)式中:y S jCSA 表示第j 个尺度S j 的CSA 输出特征,j =1,2,3,4;F S j Si(⋅)表示将尺度为S i 的特征图采样到尺度为S j 的特征图.同时,在该模块中引入短连接以改y 模块A1/161/321/81/4卷积层残差组平均池化跨尺度聚合(CSA )y ResGroupy S 2CSAy S 3CSAy S 4CSAy S 1CSA逐元素相加LeakyReLU-1×1Conv-LeakyReLU-1×1Conv-LeakyReLU-UpSample 3×3Conv-y CSACat级联Cy CSAEBlock图7CSAE Block 结构Fig.7CSAE block129湖南大学学报(自然科学版)2022年善其梯度流.综上所述,CSAE Block 总的输出表达式为:ìíîïïïïy CSACat =Cat j =14()F up ()LeakyReLU 0.2()Conv 11()y S jCSAy CSAE Block =Conv 13()Cat ()y CSACat ,y ResGroup (17)其中y CSACat 表示对上采样为统一大小的输出特征进行级联操作.1.6损失函数为了获得更好的去雾效果,本文使用3个损失函数(有图像去雾损失L rh 、边缘损失L edge 以及对比度增强损失L ce )作为网络训练的总损失L total ,即:L total =αL rh +γL edge -λL ce(18)其中:α,γ和λ均为任意非负常数.1)图像去雾损失函数.本文将L 1损失和L 2损失进行简单结合,构成车牌图像去雾损失函数:L rh =1N ∑i =1N ()I i gt -F Net ()I i haze 1+I i gt -F Net ()I ihaze 2(19)式中:N 表示输入图像像素数;I gt 为干净图像;I haze 为车牌雾图;F Net (⋅)表示车牌去雾网络函数.一方面通过L 1损失函数来获得较高的PSNR 和SSIM 值,另一方面则通过L 2损失函数来尽可能提高去雾图像的保真度.2)边缘损失函数.为了加强输出去雾图像的边缘轮廓信息,本文利用Sobel 边缘检测算法来获得车牌去雾图像和干净图像的边缘轮廓图,分别为E FNet()I haze和E I gt,计算两者的L 1范数,获得边缘损失函数:L edge=E FNet()I haze-E I gt1(20)3)对比度增强损失.为了提高车牌去雾图像的颜色对比度,本文最大限度地提升每个单独颜色通道的方差,即最大化如下表达式:L ce=(21)式中:x 表示图像的像素索引;FˉNet (I haze )为去雾网络输出图像F Net (I haze )的平均像素值.值得注意的是,所期望的输出去雾图像应该增强其对比度,所以L ce 需要最大化,因此在总损失L total 中应减去该项.2训练与测试2.1车牌雾图数据集(LPHaze Dataset )的制作为了解决车牌去雾网络训练过程中缺失的车牌雾图数据集问题,受RESIDE 数据集制作方法的启示,本文采用成熟的ASM 理论来进行车牌雾图数据集的制作.车牌图像数据主要来源于OpenITS 提供的OpenData V3.1-SYSU 功能性能车牌图像数据库,并以中科大开源数据集CCPD [22]作为补充,具体制作方法如下:1)预处理.从OpenITS 和CCPD 数据集中随机选取2291张清晰图像,并对这些清晰车牌图像的车牌区域进行截取;2)配置大气散射模型参数值.参照RESIDE 数据集所选取的参数值范围,随机选取如下一组大气光值A =[0.6,0.7,0.8,0.9,1.0]和一组大气散射系数值β=[0.4,0.6,0.8,1.0,1.2,1.4,1.6],并将场景深度d (x )置为1;3)合成车牌有雾-无雾图像对.采取一张清晰车牌图像对应多张车牌雾图的方法来合成图像对,即根据大气散射模型,结合步骤2中选定的参数值,以一张车牌无雾图像对应35张有雾图像的方法来合成数据集.合成车牌雾图示例如图8所示.(a )原图(b )(A =0.6,β=0.4)(c )(A =0.7,β=0.8)(d )(A =0.8,β=1.2)(e )(A =1.0,β=1.6)图8合成车牌雾图示例Fig.8Example of fog map of composite license plate4)划分训练集和验证集.训练集中干净车牌图像共1697张,对应的车牌雾图共59395张;验证集中干净图像共594张,对应车牌雾图共20790张.2.2实验设置本文采用自制的车牌雾图数据集(LPHaze Data⁃130第6期陈炳权等:基于深度多级小波U-Net 的车牌雾图去雾算法set )作为车牌图像去雾网络的训练和验证数据,其中所有图像像素大小均设置为64×128×3,batch size 为64.并对训练数据进行数据增强操作:随机水平翻转和随机垂直翻转(翻转概率随机取值0或1),以提升网络的鲁棒性.此外,在训练过程中,使用Adam 优化器来优化网络,其参数均采用默认值(β1=0.9和β2=0.999),并通过梯度裁剪策略加速网络收敛,训练800个epoch ,初始学习率为1e -4,总损失函数中α=1、γ=0.1和λ=0.01.采用Pytorch 包进行车牌雾图去雾网络结构代码的编写和训练,整个训练过程均在NVIDIA Tesla T4的显卡上运行.实验主要包括两个部分:其一,测试本文提出的车牌雾图去雾网络模型,其二,对其进行消融实验.上述实验在合成车牌雾图和自然拍摄的车牌雾图上进行测试,所有测试图像均随机选自OpenITS 提供的车牌图像数据库(与LPHaze Dataset 训练集中的数据不重合),并从测试结果中选取如下5种组合类型进行定性和定量分析,分别为(A =0.6,β=0.8)、(A =0.7,β=1.0)、(A =0.8,β=1.2)、(A =0.9,β=1.4)和(A =1.0,β=1.6),同时对其依次编号为组合A 到E.3结果与分析3.1测试实验1)合成车牌雾图去雾结果为了进一步评估算法性能,将本文算法与最近出现的经典去雾算法(基于引导滤波器的暗通道先验算法[4](GFDCP )、基于深度学习的端到端的去雾网络[8](DehazeNet )、端到端的一体化去雾网络(AODNet )、端到端门控上下文聚合去雾网络[23](GCANet )和端到端特征融合注意力去雾网络[17](FFANet ))进行比较.以上算法统一在LPHaze Data⁃set 的验证集上进行测试,并选取其中5类合成车牌雾图的测试结果进行实验分析,其结果见表2.由表2可以看出,在5类不同大气光和散射系数的合成车牌雾图上,与GCANet 得到的结果相比,在PSNR 均值上分别提高了5.64、6.74、8.84、10.52、11.88dB ,SSIM 均值上则分别提高了0.0368、0.0599、0.0991、0.1496、0.2225.同时,在图9中的PSNR 和24222018161412108P S N R (d B )组合A组合B组合C组合D组合EGFDCPDehazeNet AODNet GCANet FFANet 本文算法组合类型(a )6种算法在5类组合上的PSNR 均值曲线GFDCP DehazeNet AODNet GCANet FFANet 本文算法1.00.90.80.70.60.5S S I M组合A组合B组合C组合D组合E组合类型(b )6种算法在5类组合上的SSIM 均值曲线图96种算法在5类合成车牌雾图上的PSNR 和SSIM 均值曲线Fig.9PSNR and SSIM mean curves of 6algorithms on5types of composite license plate fog map表2合成车牌雾图去雾图像的PSNR (dB )/SSIM 均值Tab.2PSNR (dB )/SSIM mean of defogging image of composite license plate fog image组合类型(A =0.6,β=0.8)(A =0.7,β=1.0)(A =0.8,β=1.2)(A =0.9,β=1.4)(A =1.0,β=1.6)GFDCP20.75/0.946119.23/0.924817.85/0.900715.63/0.861612.70/0.8035DehazeNet19.31/0.895216.92/0.846014.20/0.793611.71/0.74509.57/0.6882AODNet13.79/0.775715.12/0.801314.60/0.745211.64/0.64818.01/0.5349GCANet18.86/0.925516.31/0.890613.82/0.845911.92/0.791310.14/0.7091FFANet18.09/0.894718.65/0.878419.31/0.851212.76/0.71678.61/0.5407本文算法24.50/0.962323.05/0.950522.66/0.945022.44/0.940922.02/0.9316131湖南大学学报(自然科学版)2022年SSIM 均值曲线图中亦可知,在重构图像质量方面,本文算法在处理不同雾度的合成车牌雾图上明显优于上述5类经典算法.最后,从合成车牌雾图的去雾图像中选取部分图片进行效果展示,如图10所示.从去雾效果中可以直观感受到,本文算法相较于其它算法而言,具有较少的雾度残留以及颜色失真.2)自然车牌雾图去雾结果本文还对实际拍摄的自然车牌雾图进行测试,并与上述5种经典算法的去雾效果进行视觉比较.该测试数据选自OpenITS 的车牌图像数据库,共915张实际拍摄的车牌雾图,视觉对比结果如图11所示.从图11可知:在处理常见的蓝底车牌雾图时,本文算法很少出现过度曝光和图像整体偏暗的问题,且雾度残留也很少;对于其它底色的车牌雾图(如图11中的黄底和蓝底车牌),本文算法在去雾效果上相较于上述5种经典算法仍能保持自身优势,并且在颜色、字符等图像信息上也能得到较好的恢复.(a )(A =0.6,β=0.8)(b )(A =0.7,β=1.0)(c )(A =0.8,β=1.2)(d )(A =0.9,β=1.4)(e )(A =1.0,β=1.6)合成雾图GFDCPDehazeNetAODNetGCANetFFANet本文算法干净图像图10合成车牌雾图去雾效果展示Fig.10Fog removal effect display of composite license plate图11实际拍摄的车牌雾图去雾效果展示比较Fig.11Comparison of defogging effect of actual license plate fog map自然车牌雾图GFDCP DehazeNet AODNet GCANet FFANet 本文算法132第6期陈炳权等:基于深度多级小波U-Net的车牌雾图去雾算法3.2不同模块对网络性能的影响为了分析其中各个模块的重要性,本文在LP⁃Haze Dataset数据集上进行消融研究分析,以基于ResGroup和“SOS”Block的MWCNN去雾网络作为基准网络模块,对于其他不同的网络模块,其简化形式及说明如下,R1:基于ResGroup和“SOS”Block的MWCNN作为基准网络,该网络不包含DCPA Block、LMSA和CSAE Block;R2:具有DCPA Block的基准网络;R3:具有DCPA Block、LMSA和CSAE Block的基准网络,即最终的车牌雾图去雾网络.上述网络模块均只训练150个epoch,且初始学习率均为1e-4,并在LPHaze Dataset的验证集上进行测试,其测试结果如表3所示.表3不同网络模块在LPHaze Dataset的验证集上测试结果的均值Tab.3Mean value of test results of different networkmodules on the verification set of LPHaze Dataset网络R1 R2 R3“SOS”Block√√√DCPABlock√√LMSA√CSAEBlock√PSNR/dB22.4722.4323.27SSIM0.94210.94320.9513由表3可得,在不加入DCPA Block、LMSA和CSAE Block的情形下,PSNR和SSIM的均值分别可以达到22.47dB和0.9421,而在加入三者后,PSNR 和SSIM均值则分别提升了0.8dB和0.0092,从而使网络能重建出高质量的去雾图像.3.3不同损失函数对网络性能的影响为了分析损失函数的有效性,本文算法分别采用L1、L2、L rh(即L1和L2损失的简单结合)和L total这四类损失函数来进行网络模型的训练,训练150个ep⁃och,且初始学习率为1e-4.分别在LPHaze Dataset的验证集上进行PSNR和SSIM的指标测试,其实验结果如表4所示.从表4可知,只使用L rh损失函数时,表4不同损失函数下车牌去雾网络测试结果Tab.4Test results of license plate defogging networkunder different loss functions损失函数L1L2L rhL total PSNR/dB22.7422.1923.0623.27SSIM0.94170.93710.94710.9513平均PSNR和SSIM可达到23.06dB和0.9471,且相较于单独使用L1或L2时均有着明显提升.而使用总损失函数L total时,平均PSNR和SSIM分别提升了0.21dB和0.0042,从而使网络性能得到较大的改善.4结论本文提出了一种基于深度多级小波U-Net的车牌雾图去雾算法,该算法以MWCNN作为去雾网络主体框架.首先,为了在小波域和空间域中尽可能地整合不同层级和尺度的图像特征,引入了“SOS”增强策略,并在MWCNN中间进行跨层连接,以此对图像特征进行整合、完善和优化;其次,本文将像素注意力、通道注意力和离散小波变换进行有效融合,从而尽可能去除车牌雾图中的雾度;最后,通过跨尺度聚合增强模块来弥补小波域和空间域之间存在的图像信息差异,进一步提高了重构图像质量.自然车牌雾图和LPHaze Dataset的验证集上的实验结果表明,在处理具有不同大气光照和雾度的车牌雾图上,本文算法具有较好的去雾表现,并且在处理具有不同底色的车牌雾图时具有一定的优势.参考文献[1]YADAV G,MAHESHWARI S,AGARWAL A.Foggy image en⁃hancement using contrast limited adaptive histogram equalizationof digitally filtered image:performance improvement[C]//2014In⁃ternational Conference on Advances in Computing,Communica⁃tions and Informatics(ICACCI).September24-27,2014,Delhi,India.IEEE,2014:2225-2231.[2]RUSSO F.An image enhancement technique combining sharpen⁃ing and noise reduction[J].IEEE Transactions on Instrumenta⁃tion and Measurement,2002,51(4):824-828.[3]GALDRAN A,BRIA A,ALVAREZ-GILA A,et al.On the dual⁃ity between retinex and image dehazing[C]//2018IEEE/CVF Con⁃ference on Computer Vision and Pattern Recognition.June18-23,2018,Salt Lake City,UT,USA.IEEE,2018:8212-8221.[4]HE K,SUN J,TANG X.Guided image filtering[C]//DANIILIDISK,MARAGOS P,PARAGIOS puter Vision-ECCV2010,11th European Conference on Computer Vision,Herak⁃lion,Crete,Greece,September5-11,2010,Proceedings,Part I.Springer,2010,6311:1–14.[5]HE K M,SUN J,TANG X O.Single image haze removal usingdark channel prior[C]//IEEE Transactions on Pattern Analysisand Machine Intelligence.IEEE,:2341-2353.[6]ZHU Q S,MAI J M,SHAO L.A fast single image haze removal133。
检测一氧化氮的荧光探针研究进展
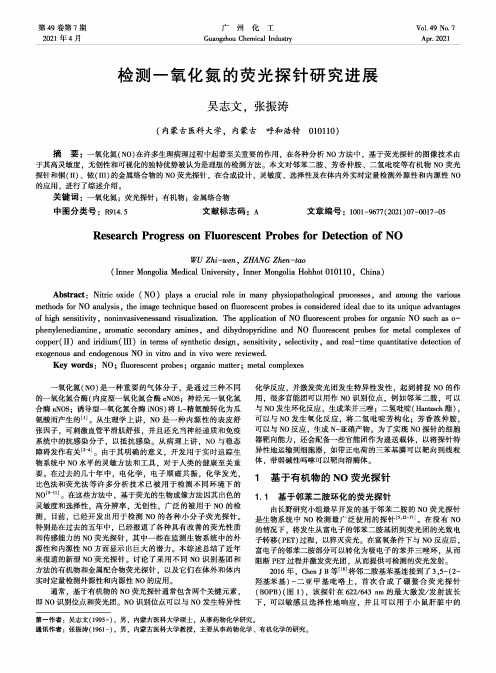
第49卷第7期2021年4月广州化工Guangzhou Chemical IndustryVol.49No.7Apr.2021检测一氧化氮的荧光探针研究进展吴志文,张振涛(内蒙古医科大学,内蒙古呼和浩特010110)摘要:一氧化氮(NO)在许多生理病理过程中起着至关重要的作用,在各种分析NO方法中,基于荧光探针的图像技术由于其高灵敏度,无创性和可视化的独特优势被认为是理想的检测方法。
本文对邻苯二胺、芳香仲胺、二氢毗睫等有机物NO荧光探针和铜(II)、铁(III)的金属络合物的NO荧光探针,在合成设计、灵敏度、选择性及在体内外实时定量检测外源性和内源性NO 的应用,进行了综述介绍。
关键词:一氧化氮;荧光探针;有机物;金属络合物中图分类号:R914.5文献标志码:A文章编号:1001-9677(2021)07-0017-05 Research Progress on Fluorescent Probes for Detection of NOWU Zhi-wen,ZHANG Zhen-tao(Inner Mongolia Medical University,Inner Mongolia Hohhot010110,China)Abstract:Nitric oxide(NO)plays a crucial role in many physiopathological processes,and among the various methods for NO analysis,the image technique based on fluorescent probes is considered ideal due to its unique advantages of high sensitivity,noninvasivenessand visualization.The application of NO fluorescent probes for organic NO such as o-phenylenediamine,aromatic secondary amines,and dihydropyridine and NO fluorescent probes for metal complexes of copper(II)and iridium(III)in terms of synthetic design,sensitivity,selectivity,and real-time quantitative detection of exogenous and endogenous NO in vitro and in vivo were reviewed.Key words:NO;fluorescent probes;organic matter;metal complexes一氧化氮(NO)是一种重要的气体分子,是通过三种不同的一氧化氮合酶(内皮型一氧化氮合酶eNOS;神经元一氧化氮合酶nNOS;诱导型一氧化氮合酶iNOS)将L-精氨酸转化为瓜氨酸而产生的⑴。
太子参中环肽Pseudostellarin

太子参中环肽Pseudostellarin天然产物研究与开发NatProdResDev2007,19:206-210,239文章编号:1001-6880(2007)02-0206-06太子参中环肽PseudostellarinB含量HPCE测定及其指纹图谱研究李文龙,陈军辉,韩超.,王小如¨国家海洋局第一海洋研究所现代分析技术及中药标准化重点实验室,青岛266061;中国海洋大学化学化工学院,青岛266003;厦门大学化学化工学院化学系现代分析科学教育部重点实验室,厦门361005摘要:建立了测定太子参中环肽PseudostellarinB含量的高效毛细管电泳(HPCE)分析方法,对lO种不同产地太子参中环肽PseudostellarinB的含量进行了测定.HPCE工作条件:采用未涂层熔融石英毛细管(内径75txra,有效长度50cm),分离电压为l5kV,柱温2O℃,二极管阵列检测器(DAD)检测波长为203nm,缓冲液为2Ommol/L.硼砂(pH=9.3)溶液.在选定的工作条件下,环肽PseudostellarinB浓度与其响应信号值之间具有较好的线性相关性(Y=0.6357X+2.546,R=O.9985),加标回收率在93.8%一105.6%之间.在此基础上进行了太子参HPCE指纹图谱研究,采用中药指纹图谱相似度计算软件对不同太子参样品的HPcE指纹图谱进行相似度计算,以系统生成的对照指纹图谱为对照模板,lO份样品中有8份的相似度在0.90以上,说明该方法可用于太子参质量控制.关键词:太子参;环肽PseudostellarinB;高效毛细管电泳法;指纹图谱中图分类号:R284.2文献标识码:A DeterminationofPseudostellarinBinPseudostellariaheterophyllaby HPCEandStudyonItsFingerprintsAnalysisLIWen—long,CHENJun—hui一,HANChao,W ANGXiao2ru' QingdnoKeyLaboratoryofAnalyticalTechnologyDevelopmentandStandardizationofCh ineseMedicines,FirstInstituteOceanographyofSOA,Qingdao266061,China;CollegeD,ChemistryandCh emicalEngineering,OceanUnive~i@ofChina,Qinadao266006,China;DepartmentofChemistryandtheKeyL aboratoryofAnalyticalScienceoft^eMOE.CollegeofChemistryandChemicalEngineering,XiamenU niversity,Xiamen361005,ChinaAbstract:AmodemHPCEanalysismethodwasdevelopedfordeterminatingcychcpepfideP seudostellarlnBinPseudos~llariaheterophylla(Miq.)Pax.SeparationanddeterminationwerecarriedoutbyH PCEunderthefollowingconditions:baredfusedsilicacapillary(50amX75txrai.d.),20mmoL/Lborate(pH=9.3)asb uffer,thelUllvoltageis+15kV.detectionlengthofUV at203am,andcolumntemperatureof20℃.There8ultsindic atedthattlledevelopedmethodwassimple.accurateandreliableforthedeterminationofPseudostellarinBwithagoo dlinearlty(Y=0.6357X+2.546,R=0.9985),andtherecoveryrangesof93.8%一105.6%.Basedonthismethod,n1eHPCEfingerprintsof Pseudastellariaheterophylla(Miq.)Paxwereestablished.ThefingerprintsWerecomparedb ythesoftwareofthesimilar- ityevaluationsystemforchromatographicfingerprint.Thefingerprintcongruencecoefficie ntsof8electmphemgramsin10wereabove0.90.ThemethodofHPCEfingerprintisreliableandaccurate.Keywords:Pseudostellariaheterophylla(Miq.)Pax;PseudostellarlnB;HPCE;fingerprint太子参又名孩儿参,童参,为双子叶植物药石竹科(Caryophyllaceae)植物异叶假繁缕属植物Pseudostellariaheterophylla(Miq.)Pax的块根.具有收稿13期:2006-08—16接受13期:2006-09-30基金项目:国家自然科学基金项目(20235020);国家科技部攻关项目(2003BA759C)+通讯作者Tel:86_532.88963253;E—mail:***************.ca补益脾肺,益气生津的功效,主治肺虚咳嗽,脾虚食少,心悸,怔忡等¨J.环肽是指氨基酸以酰胺键互相连接而形成的环状化合物,生物活性环肽能形成限制性构象,与相应线性肽相比在生物体内具有更好的抗酶解和抗化学降解的能力.最近几年发现石竹科环肽有较广泛的生物活性驯.2005版《中国药典》中还没有明确太子参的指标性成分以及太子李文龙等:太子参中环肽PseudostellarinB含量HPCE测定及其指纹图谱研究参质量控制方法.中药色谱指纹图谱是一种综合的,可量化的色谱鉴定手段.借以鉴别真伪,评价原料药材,半成品和成品质量均一性和稳定性.其基本属性是"整体性"和"模糊性".在有效成分不完全明确的前提下,制定中药材的指纹图谱,对有效控制中药材的质量,具有重要意义'.目前国内仅有一篇文献利用HPLC对太子参进行指纹图谱研究J,但是该文对各指纹峰均未进行定性定量分析.HPCE是一种基于不同质荷比的离子在同一电场下迁移速度不同的分离手段,具有分离效率高,分析速度快等特点,已成为除GC,HPLC之外进行复杂样品分离分析的一种新的选择,目前广泛用于中药中有效成分的测定及中药材指纹图谱研究..国内外利用HPCE定量测定太子参环肽Pseudostel- latinB及太子参HPCE指纹图谱研究尚未见有文献报道.本文探索采用HPCE对太子参药材进行活性成分测定及其指纹图谱研究,以本实验室分离,纯化所得的环肽PseudostellarinB纯品为对照品,建立了高效毛细管电泳法测定太子参中环肽Pseudostella- nB的方法;并在此基础上得到了太子参的HPCE指纹图谱,对所得指纹图谱进行了相似度计算,结果满意.1实验部分1.1仪器与试剂美国Agilent公司G1062A型HPCE仪(配有二极管阵列检测器(DAD)),未涂层熔融石英毛细管(内径75Ixm,有效长度50cm),SK200LH型超声波仪(上海科导超声仪器有限公司),旋转蒸发仪R201(上海申生科技有限公司).硼砂(分析纯),1mol/LY aOH溶液,甲醇(优级纯),乙酸乙酯(优级纯),实验用水为Milli-Q超纯水. 太子参环肽PseudostellatinB对照品由本实验室分离纯化所得(含量测定用).所有试剂溶液及试样进入HPCE系统前均经过0.2m微孔滤膜过滤.本文所采用的太子参样品:福建,江苏,贵州,湖南产太子参由厦门倍尔思生化科技有限公司提供; 山东,浙江,安徽产太子参购于药材公司.1.2HPCE工作条件采用压力进样方式,压力50bar,进样时间5S,分离电压15kV,紫外检测波长203nm,带宽8nm,参比波长450nm,带宽20nm.运行缓冲液为2O mmol/L的硼砂溶液(用1mol/L的NaOH溶液调pH值至9.3),试样进入毛细管前,均需过0.2微孔滤膜过滤,分析开始前,依次用1mol/LNaOH, 超纯水和缓冲液冲洗毛细管各20min,分析过程中, 及时更换缓冲液瓶中的缓冲溶液,以保证两缓冲液瓶中缓冲液组分一致,保证分析精度.1.3对照品及供试品溶液制备准确称取10.0mg环肽PseudostellatinB对照品,放入10mL容量瓶中,用超纯水溶解定容,制成1g/L的储备液.供试样品(粉碎,过6O目筛)在5O℃烘箱中干燥12h,准确称取1.0g,以甲醇为溶剂,超声辅助提取3次(3×10mE),每次提取60min.合并各次提取液过滤,滤液经旋转蒸发仪浓缩至干,用超纯水溶解定容到10mL容量瓶中,过0.20Ixm滤膜后作为供试品溶液.2结果与讨论2.1提取方法及HPCE分离条件优化2.1.1不同提取方法对提取效率的影响本文采用了回流法和超声辅助提取两种方法对样品进行提取,结果显示,回流法提取效率稍高于超声法,后者约为前者的95%,相差不大.回流法操作复杂,且加热过程可能会引起其它组分的变化,而超声法方便快速,因而选用超声法进行提取.以PseudostellarinB的提取率为指标,检验了3种提取溶剂:超纯水,乙酸乙酯和甲醇的提取效率(因后两者在环肽PseudostellatinB的检测波长203 nm处有吸收,干扰了其测定,因此必须将其提取液旋转蒸干后用超纯水重新溶解进行进样测定),结果表明,甲醇的提取效率最高,超纯水和乙酸乙酯的提取效率分别为甲醇的55%和7l%,故本文采用甲醇作为提取溶剂.2.1.2缓冲液浓度及pH值和分离电压对分离过程的影响对HPCE分离过程的中的三个参数缓冲液浓度及pH值和分离电压进行正交实验设计,结果表明: 随着硼砂溶液浓度的升高,样品中各峰的保留时间差别逐渐增大,分离效果也越好,但浓度过高, 噪音也明显增大,电流显着增加,产生热量较多,不利于分离,因此选择20mmol/L的硼砂溶液作为缓冲液,各峰得到较好的分离.太子参环肽PseudostellatinB在较高pH值的缓冲溶液中完全电离成阴离子,能够有效抑制管壁吸天然产物研究与开发附,离子在分离过程中由正极向负极泳动,与电渗流速度相同,其表观速度为电泳速度与电渗流速度的矢量和,二者方向相同,有利于增加分析速度,缩短每次进样分析的周期,pH为9.3时,分离效果较好, 且分析时间较短.在1O一30kV的范围内,电压越高,分析速度越快,但在较高电压下,分离效果及峰形较差,且噪音明显增大,不利于分析,因而选用较为适中的15kV 作为分离电压,效果较好.2.I.3检测波长的选择由于太子参环肽PseudostellarinB中不存在发色团,只是在紫外末端有较大吸收,且随着检测吸收波长的增大,样品吸收急剧下降,见图1,本文选用203 nm作为检测波长,仪器易于稳定,基线平稳,噪音较小,有利于太子参环肽PseudostellarinB的检测.20o250300350400图1太子参环肽PseudostellarinB的DAD扫描光谱图Fig1DADscanspectrumofPseudostellarinBpeak2.1.4样品分离效果采用优化后的提取方法和HPCE工作条件,测定对照品溶液和供试品溶液,比较太子参环肽PseudosteUarinB对照品溶液及供试品溶液液相色谱图(见图2),可以看出在1.2所述的仪器工作条件下色谱峰峰形较好,可准确测定其峰面积及峰高并进行定量分析.24681012时间Time(rain)图2对照品和供试品的HPCE色谱图Fig.2EleetropherogramofPseudostellarlnBstandardand extractofPseudostellariaheterophylla(Miq.)Pax2.2标准曲线的绘制吸取一定量的对照品储备液,用超纯水稀释,配制成1,5,1O,15,2O,25,30mg/L的标准溶液.按照1.2所述工作条件分别进样分析,测定太子参环肽PseudostellarinB的峰面积,以峰面积为横坐标,对照品浓度(mg/L)为纵坐标,绘制标准曲线.环肽PseudostellarinB的线性回归方程及相关系数为:y=O.6357X+2.546,R=0.9985.结果表明,在1—30mg/L范围内,浓度与峰面积呈良好线性关系.2.3方法学考察2.3.1精密度试验按照1.2HPCE工作条件,对环肽Pseudostella—rinB对照品溶液进行6次重复进样分析,测得其峰面积和保留时间,分别计算其标准偏差,峰面积和保留时间RSD值分别为0.32%和0.18%,表明仪器精密度良好.2.3.2稳定性试验将供试品溶液按照1.2HPCE工作条件,分别在O,2,4,8,12,24,36,48h时进样测定,结果显示供试品溶液中环肽PseudostellarinB在48h内峰面积和保留时间无明显变化,峰面积和保留时间的RSD值分别为1.21%和0.46%,说明供试品溶液在48h内化学性质稳定.2.3.3重现性试验精密称取同一样品五份,按1.3处理方法制成供试品溶液,按照1.2的HPCE工作条件进样测定, 测得环肽PseudostellarinB峰面积和保留时间,并计算其标准偏差,峰面积和保留时间的RSD值分别为2.55%和0.62%,表明该方法重现性良好.2.3.4回收率试验精密称取1.0g已知环肽PseudostellarinB含量的太子参样品5份,定量加人对照品,按1.3处理方法将其制成供试品溶液,按1.2的HPCE工作条件测定加标回收率,每份样品平行进样3次,结果取平均值,并计算RSD%,结果见表1.结果表明,本法回收率高,测定结果准确,方法可行.2.3.5色谱峰的定位本文主要根据对照品的保留时间对样品进行色谱峰定性,实验中以适量的标准储备液加人样品溶液中,通过对比各峰的吸光度,确认吸光度明显增大的峰为对照品峰,并将其uV谱图与相应已知标准品图谱进行比较印证,以确定与对照品相应的峰位.李文龙等:太子参中环肽PseudostellarinB含量HPCE测定及其指纹图谱研究209 表1太子参环肽PseudostellarinB加样回收率实验结果(Tt=3)Table1RecoverytestoftheaddedPseudostellarinBstandard(Tt=3)2.3.6环肽PseudosteUarinB的检测限将样品逐级稀释进样,测定其峰高响应值及基线噪音强度,以3倍信噪比计算其检测限,结果表明,在选定的仪器工作条件下,环肽PseudostellarinB检测限达到0.6mg/L.2.4不同产地太子参样品中环肽PseudostellarinB的含量测定结果取不同产地太子参,按1.4处理方法将其制成供试品溶液,按1.2HPCE工作条件,测定其中太子参环肽PseudostellarinB的含量,每批样品进行了三次平行测定,结果见表2.可见不同产地太子参样品中环肽PseudostellarinB含量差异较大,5号太子参样品(安徽产)环肽PseudostellarinB含量最高,而湖南和山东产太子参中环肽PseudostellarinB含量较低,样品中环肽PseudostellarinB的平均含量为158.43g.表2不同太子参样品中环肽PseudostellarinB的含量(n=3)Table2COntentsofPsel】dostellarinBinPseudostellariahetero—phylla(Miq.)PaxcoHectedfromdifferentregions(n=3)2.5不同太子参样品指纹图谱的建立按照暂行的中药注射剂指纹图谱研究的技术要求,制定HPCE指纹图谱所需的技术参数主要有三个:一,必须根据参照物的迁移时间,计算指纹峰的相对保留时间,标定中药材的共有指纹峰;二,以参照物的峰面积作为1,计算各共有指纹峰面积与参照物峰面积的比值,各共有指纹峰面积比值必须相对固定;三,中药材供试品的图谱与指纹图谱比较,非共有峰总面积不得大于总峰面积的10%.本文对1O批次太子参甲醇提取物在1.2HPCE工作条件下进样分析,每个样品进行三次平行分析, 其色谱图共有l1个共有峰,其峰面积总和占总峰面积的98%以上,其中2号峰确认为太子参环肽PseudostellarinB,选择该峰作为参照峰,分别求出各特征峰的相对保留时间(仅)和相对峰面积.40302010***********时问Time(min)图3太子参甲醇提取物的共有指纹图谱Fig.3MutualfingerprintsofextractofPseudostellariahet—erophflla(Miq.)Pax2.6指纹图谱相似度分析在化学计量学中,常采用相关系数和相合系数(矢量间的夹角余弦)作为评价指纹图谱相似度的度量l1引,浙江大学制药工程研究所计算机辅助中药分析实验室研发的"中药指纹图谱相似度计算软件"采用夹角余弦作为相似度的评价指标,是国家药典委员会推荐使用的指纹图谱相似度计算软件引.本文采用该软件对测定结果进行图谱比对,评价供试样品指纹图谱与系统生成的对照指纹图谱(各样品谱图峰面积的平均值)的相似性,对照共有图计算得到1O批太子参药材提取物的相似度,结果见表4.从表4可见,除8号样品与1O号样品外,其他8批样品色谱图的相似度均在0.90以上,从太子参环肽PseudostellarinB的测试结果来看,8号样品与1O号样品的含量也较低,这在相似度的计算结果中得到了反映.210天然产物研究与开发V o1.190.83911.1351.21.4161.6461.7531.871.9562.2l12.320.340.0oO.62O.730.84O.89 1.61 1.64 1.15 1.36 O.85 1 O.O7 0.22 O.2 O.240.271.45 O.8l 0.15 O.11 0.71 1 O.O8 0.41 O.24 0.77 0.34 O.93 O.76 0.14 O.12 O.33 1O.55 O.11 O.2 O.27 0.46 0.22 O O.16 O.33 1 0.11 O.55 O.12 O.25 O.27 0.48 0.15 O.13 O.15 O.33 1 O.15 O.6 0.19 O.29 O.33 0.46 0.18 O.190.52 1 O.O5 O.69 O.36 0.09 O.16 1.o6 1.04 0.54 0.o6 O.31 1 O.16 0.22 O.36 O.36 O 1.27 l O.25 O.23 O.58 1 0.05 O.59 O.38 0.09 O.191.I1O.59O.o71.0910.153.1O.33O.29O.31.480.94O.19O.25O.291O0.10.09O.730.262.170.3l0.16O.22表41O批太子参样品提取物指纹图谱相似性分析结果Table4Similarityanalysisresultofextractsof10Pseudostel—lariaheterophylla(Miq.)PaxN0.12345678910似度o.9230.951o.9240.9480.9330.9820.9170.7760.9400.829~imflanty 目前,中药材的分类及鉴别在很大程度上仍依赖于经验和表观分析,缺乏从定性定量分析数据中获取评价依据的统一,实用的方法.相似度计算方法数学概念简单,数据处理过程简便,快速,在定量计算色谱指纹图谱相似度方面有其优势.在指纹图谱相似度计算中,一般将所选取的色谱峰同时作比较计算,反映指纹图谱的整体相似性,可对中药产品质量做出较全面,准确的评价.本文以不同太子参样品的平均峰面积作为对照模板,进行相似度计算,优点是能较全面反映不同太子参样品的整体特征.如果利用多种道地药材的标准提取物建立了标准谱图,则通过计算待测样品与标准谱图的相似度,可以对样品质量进行正确评价,这也是今后进一步研究的目标.3结论本文所建立的太子参中环肽PseudostellarinB含量HPCE测定的方法前处理便易操作,分析速度快,具有良好的重珊陛,稳定性,可作为太子参中环肽PseudostellarinB含量的检测方法及用于太子参产品的质量控制.在此基础上所建立的太子参中环肽PseudostellarinBHPCE指纹图谱研究方法分析时间短,提取物中共有峰数目较少,便于进行质量控制,将其用于实际的提取物鉴定工作中,方法快捷,结果可靠.参考文献1LiSH(李仕海),LiuXH(刘训红).Analysisofaminoacid andtraceelementinradixpseudostellariaegrowninJiangsuprovince.LishizhenMedMaterMedRes(时珍国医国药),2001,12:199-20o.2I(irkRG,RaymondCS,JrLewisKP,eta1.CirculinsAandB.Novelhumanimmunndeficiencyvires(HIV).inhibitory macrocyclicpeptidesfromthetropicaltreeClm~aliap口【n7 f.JAmChemSoc,1994,116:9337-9338.3Y aharaS,ShigeyamaC,NoharaT.Structuresofanti—aceand renninpepfidesfromLyciiradicisCOl'reX..TetrahedronLett, 1989,30:6041-6042.4MoritaH,YunYS,TakeyaK,eta1.Conformationalanalysis ofacyclehexapeptide,segetalinAfromV accariasegetalis. Tetrahedron,1995,51:5987-6002.5HiroshiM,TakashiK,HideyukiK,eta1.PseudostellarinsA—C,newtyrosinaseinhibitorycycHcpeptidesfromPseudostel- lariaheterophylla.Tetrahedron,1994,50:6797-68046XiePS(谢培山).Afeasiblestrategyforapplyingchromatog- raphyfingerprinttoassessqualityofChineseherbalmedi—cine.Gil,~lgzholl:TheProceedingsofInternationalSymposi一啪ofHerbalDrugQualityAssessmentbyChromatographic Fingerprint,2001.7GongF,LiangYZ,XiePS,rmationtheoryapplied tochromatographicfingerprintofherbalmedicineforquality contro1.JChromatogrA,2003,1002:25-40.(下转第239页)123456789加nV0L19LI13netal:IndoleAlkaloidsfromRauwolfiavomitoria239 2.90(1H,m,H-5),2.17(1H,m,H-6a),1.88—1.92(2H,m,H-6flandH?14a),6.64(1H,d,J=6.4Hz,H.9),6.56(1H,dd,J=8.0,6.4Hz,H一10),6.60(1H,d,J=8.0Hz,H一11),1.26(1H,d,J=12.0Hz,H一14),3.15(1H,m,H一15),1.88(1H,s,H一16),4.36(1H,s,H一17),1.66(3H,d,J=6.4Hz,H一18),5.28(1H,brd,J=6.4Hz,H一19),3.41—3.58(2H,m,H-21otandH一213),3.01(3H,s,N—CH3).The"pound15wasdeterminedtobe12.hydroxymauiensine[,. AcknowledgementsTheau~lol'$aregratefultotheir colleaguesinthegroupofAnalyticalInstrumentinthe StateKeyLaboratoryofPhytoehemistryandPlantRe—sourcesinWestChina,KunmingInstituteofBotany, ChineseAcademyofSciences,formeasuringNMRand MSdata.References1YingXX,YuHA,HuaYQ,eto2.ChineseBencao.Shanghai: ScienceandTechnologyPress,1999,6,302.3o7.2MauriceMI,WilliamEC.RootAlkalodisofRauwolfiavomi? wriaMz.ntaMed.1977,32:88.98.3YuDQ,Y angJS.AnalyticChemistryHandbook,2e?d.Beijing:ChemicalIndustryPress,1999.256-258,703-704. 4ReijaJ,MauriL.AHand"CNMRstudyofsevenAjms?line-typealkaloids.P/antaMed,1996,62:577-579.5BrunoD.GiovanniP,GiulianaSR"CNMRofsomeajma.1inealkaloids.TetrahedronLetters,1981,22:4007-4008.6DanS,ElseL,JetteC,eta1.Leishmanicidal,anfiplasmodial andcytotoxicactivityofindolealkaloidsfromCorynanthe pachyceras.PlarttaMet/,2000,66:531-536.7AttaUR,AbidaS,FarzanaN,eta1.AlkaloidsfromVincaMa. 』Dr.Phytochemistry,1995,38:1057-1061.8LuciliaK,RaquelMB,IndK,eta1.IndolealkaloidsfromRauvolfiabahiensis丸DC.(Apoeynaceae).Phytochemistry, 2002,60:315-320.9ChristianeK,PierreP,SiewKK,et口Z.Indolealkaloidsfrom Rauvolfiamedia.Phytochemistry,1986,25:1783?1784.1OI{irokoS,瞄himH.TohruH.TheH.and"C.Nuclear magnetic/~sonancespectraofHarman.Reinvestigationofthe assignmentsbyone—andtwo-dimensionalmethods.ChemP肌,1993,41:1169.1172.(上接第210页)8TangBL(唐宝莲),XinSQ(辛绍祺),CaiBC(蔡宝昌),eta1.HPLCfingerprintchromatographystudyofrad/xpseudoste- llariaeheterophfllae.JNanjingUnwTradtChinMed,NatSci(南京中医药大学,自科版),2005,21:171—172.9LiuHQ(刘慧琼),GuoSH(郭书好).Modernapparatusand fingerprintoftraditionalchinesemedicine.ActaChinMed Pharmacol(中医药),2002,30:35-38.10SunY(孙沂),SuiY(隋因),GuoT(郭涛),eta1.Studyon thefingerprintsoffloscarthamifromfifferentsourcesby HPCE.ChinPharmJ(中国药学杂志),2003,38:176-178.11WangGL(王钢力),DaiZ(戴忠),LuJ(鲁静),eta/. HPCEFingerprintsof11speciesofplantsformedicinaluse ofselaginella.ChinTraditPatMed(中成药),2002,24:137? 139.12XiePS(谢培山).ChromatographicFingerprintsofTradition- alChineseMedicine(中药色谱指纹图谱).Beijing:People's MedicalPublishshingHouse,2004.131.13LiuYs(刘永锁),MengQH(孟庆华),JiangSM(蒋淑敏), eta1.Similaritysystemtheorytoevaluatesimilarityofchrom- atographicfingerprintsoftraditionalChinesemedicine.ChinJChromatogr(色谱),2005,23:158-163.。
高碳奥氏体耐热钢晶间腐蚀行为与纳米化快速脱敏的研究进展
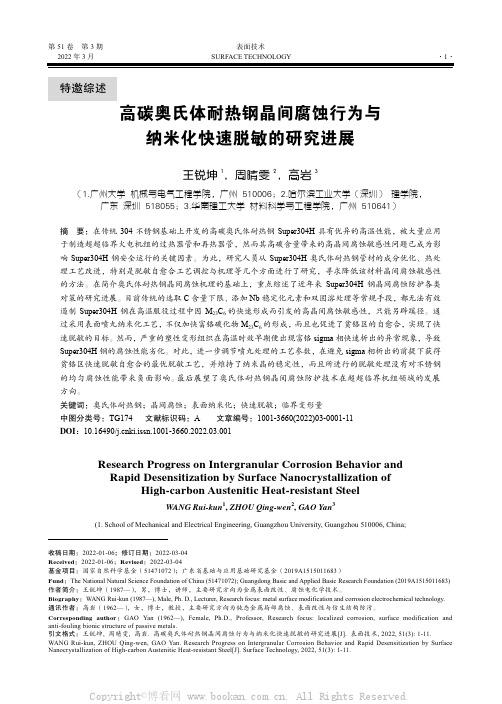
第51卷第3期表面技术2022年3月SURFACE TECHNOLOGY·1·特邀综述高碳奥氏体耐热钢晶间腐蚀行为与纳米化快速脱敏的研究进展王锐坤1,周晴雯2,高岩3(1.广州大学 机械与电气工程学院,广州 510006;2.哈尔滨工业大学(深圳) 理学院, 广东 深圳 518055;3.华南理工大学 材料科学与工程学院,广州 510641)摘要:在传统304不锈钢基础上开发的高碳奥氏体耐热钢Super304H具有优异的高温性能,被大量应用于制造超超临界火电机组的过热器管和再热器管,然而其高碳含量带来的高晶间腐蚀敏感性问题已成为影响Super304H钢安全运行的关键因素。
为此,研究人员从Super304H奥氏体耐热钢管材的成分优化、热处理工艺改进,特别是脱敏自愈合工艺调控与机理等几个方面进行了研究,寻求降低该材料晶间腐蚀敏感性的方法。
在简介奥氏体耐热钢晶间腐蚀机理的基础上,重点综述了近年来Super304H钢晶间腐蚀防护各类对策的研究进展。
目前传统的选取C含量下限、添加Nb稳定化元素和双固溶处理等常规手段,都无法有效遏制Super304H钢在高温服役过程中因M23C6的快速形成而引发的高晶间腐蚀敏感性,只能另辟蹊径。
通过采用表面喷丸纳米化工艺,不仅加快富铬碳化物M23C6的形成,而且也促进了贫铬区的自愈合,实现了快速脱敏的目标。
然而,严重的塑性变形组织在高温时效早期便出现富铬sigma相快速析出的异常现象,导致Super304H钢的腐蚀性能劣化。
对此,进一步调节喷丸处理的工艺参数,在避免sigma相析出的前提下获得贫铬区快速脱敏自愈合的最优脱敏工艺,并维持了纳米晶的稳定性,而且所进行的脱敏处理没有对不锈钢的均匀腐蚀性能带来负面影响。
最后展望了奥氏体耐热钢晶间腐蚀防护技术在超超临界机组领域的发展方向。
关键词:奥氏体耐热钢;晶间腐蚀;表面纳米化;快速脱敏;临界变形量中图分类号:TG174 文献标识码:A 文章编号:1001-3660(2022)03-0001-11DOI:10.16490/ki.issn.1001-3660.2022.03.001Research Progress on Intergranular Corrosion Behavior andRapid Desensitization by Surface Nanocrystallization ofHigh-carbon Austenitic Heat-resistant SteelWANG Rui-kun1, ZHOU Qing-wen2, GAO Yan3(1. School of Mechanical and Electrical Engineering, Guangzhou University, Guangzhou 510006, China;收稿日期:2022-01-06;修订日期:2022-03-04Received:2022-01-06;Revised:2022-03-04基金项目:国家自然科学基金(51471072);广东省基础与应用基础研究基金(2019A1515011683)Fund:The National Natural Science Foundation of China (51471072); Guangdong Basic and Applied Basic Research Foundation (2019A1515011683)作者简介:王锐坤(1987—),男,博士,讲师,主要研究方向为金属表面改性、腐蚀电化学技术。
六边形纳米CuI及其超疏水性
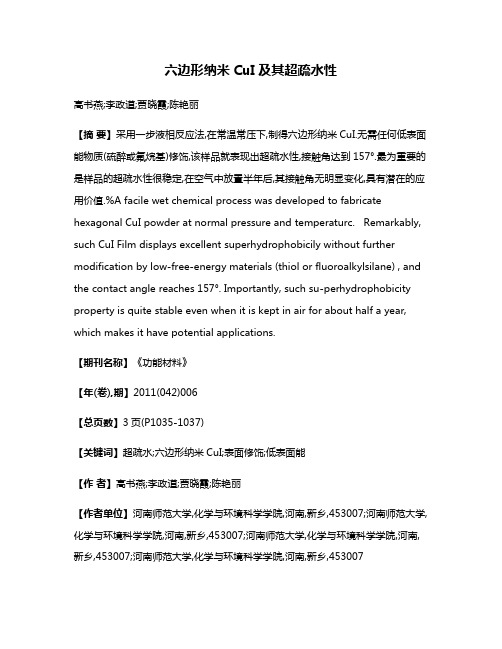
六边形纳米CuI及其超疏水性高书燕;李政道;贾晓霞;陈艳丽【摘要】采用一步液相反应法,在常温常压下,制得六边形纳米CuI.无需任何低表面能物质(硫醉或氟烷基)修饰,该样品就表现出超疏水性,接触角达到157°.最为重要的是样品的超疏水性很稳定,在空气中放置半年后,其接触角无明显变化,具有潜在的应用价值.%A facile wet chemical process was developed to fabricate hexagonal CuI powder at normal pressure and temperaturc. Remarkably, such CuI Film displays excellent superhydrophobicily without further modification by low-free-energy materials (thiol or fluoroalkylsilane) , and the contact angle reaches 157°. Importantly, such su-perhydrophobicity property is quite stable even when it is kept in air for about half a year, which makes it have potential applications.【期刊名称】《功能材料》【年(卷),期】2011(042)006【总页数】3页(P1035-1037)【关键词】超疏水;六边形纳米CuI;表面修饰;低表面能【作者】高书燕;李政道;贾晓霞;陈艳丽【作者单位】河南师范大学,化学与环境科学学院,河南,新乡,453007;河南师范大学,化学与环境科学学院,河南,新乡,453007;河南师范大学,化学与环境科学学院,河南,新乡,453007;河南师范大学,化学与环境科学学院,河南,新乡,453007【正文语种】中文【中图分类】O61;O64近年来,与水的接触角>150°的超疏水表面引起了世界范围的广泛兴趣,已经成为纳米材料和表面科学领域的热点之一[1-5]。
High temperature annealing

High temperature annealing effects on chromel (Ni90Cr10)thin filmsand interdiffusion study for sensing applicationsArindom Datta,Xudong Cheng,Michael ler,Xiaochun Li ⁎Department of Mechanical Engineering,University of Wisconsin-Madison,1513University Avenue,Madison,Wisconsin 53706,USAReceived 17October 2006;received in revised form 29October 2007;accepted 3December 2007Available online 22January 2008AbstractMetal embedded thin film thermocouples are very attractive for various applications in harsh environments.One promising technique to embed thin films micro sensors is diffusion bonding,which requires high temperatures and pressures typically in a vacuum.In this study,high temperature annealing effects on chromel (Ni90Cr10)thin film,an important sensor material as one of the components in type K thermocouple,were investigated in a diffusion bonding environment.Annealing was carried out at 800°C for one hour in a diffusion bonder under vacuum without applying pressure.Under such conditions;surface,interface and interdiffusion phenomena were investigated using different characterization techniques including X-ray Diffraction,X-ray Photoelectron Spectroscopy,Scanning Electron Microscopy,and Energy Dispersive Spectroscopy.Results indicate that the present combination of dielectrics is quite reliable and Ni90Cr10films of 500nm thickness can be used for applications at least up to 800°C due to a protective thin chromium oxide layer formation on top of the sensor film during annealing.©2007Elsevier B.V .All rights reserved.Keywords:Thin films;Chromel;Annealing;Diffusion bonding;Interdiffusion;Dielectric;Materials characterizations1.IntroductionNickel chromium (NiCr)alloys are useful owing to their potential applications as heating elements as well as in sensing [1–6].The nickel-chromium system shows that chromium is quite soluble in nickel.There is a maximum chromium composition of 47%at the eutectic temperature which drops off to about 30%at room temperature.A range of commercial alloys is based on this solid solution.Such alloys have excellent resistance to high temperature oxidation and corrosion and good resistance to wear.An addition of 20%chromium is considered the optimum for electrical resistance wires of heating elements.This composition combines good electrical properties with better strength and ductility,making it suitable for wire drawing.However,90Ni/10Cr alloy,popularly known as chromel,is also used for heating elements,and has a maximum operating temperature of 1100°C.Another important use for this alloy is in thermocouples.Chromel-Alumel (Type K)thermocoupleshave higher sensitivity than platinum based thermocouples but are much less expensive.However,the behavior of chromel thin films under high temperatures is not well understood.In this study,Ni90Cr10(chromel)thin films have been studied in detail,especially under diffusion bonding temperatures.Since metal embedded thin film thermocouples are promising for in-situ monitoring of such hostile environments [5,6],a study on chromel films would be very useful in optimizing the fabrication process for embedded sensors.Few reports were available on chromel films under high temperatures while there were some on other NiCr alloys in different compositions [2,7]and one on bulk K type thermocouple materials [8].As diffusion bonding is being studied as one of the most promising techniques for embedding thin film sensors in metals and ceramics,the survivability of thin film micro sensors at elevated temperatures is crucial for establishing a process window for sensor embedding.Various issues such as oxidation,chemical reaction and interdiffusion at elevated temperatures need to be studied.High temperature behavior of chromel thin films with respect to the underlying dielectric layers and various substrates was studied in depth.Available online at Thin Solid Films 516(2008)4307–4311/locate/tsf⁎Corresponding author.Tel.:+16082626142;fax:+16082652316.E-mail address:xcli@ (X.Li).0040-6090/$-see front matter ©2007Elsevier B.V .All rights reserved.doi:10.1016/j.tsf.2007.12.0952.Experimental detailsDepending on the experiment,chromel thin films were sputtered on either stainless steel(SS),sapphire,or silicon(Si) substrates as described later.A three-layer dielectric(Al2O3/ Si x N y/Al2O3)was grown on the SS substrate prior to the chromel deposition.This dielectric combination was optimized by the authors as the insulator for fabricating metal embedded microsensors on SS substrates[5,9].The thicknesses of Al2O3 and Si x N y films are about0.70μm and1μm respectively.Al2O3 was evaporated in an electron beam evaporation unit where a deposition rate of18–24nm/min was achieved at a working pressure of about6.66×10−3Pa with a substrate temperature at 200°C and O2backfill.The base pressure was usually on the order of10−5Pa.Si x N y was deposited by plasma enhanced chemical vapor deposition(PECVD)at300°C using SiH4,NH3 (both diluted in N2)and N2O at113Pa of chamber pressure at a deposition rate of33nm/min.A thin(50nm)chromium(Cr) layer was sputtered onto the cleaned SS substrate to promote substrate adhesion to dielectric rge pads(at least 0.5cm in one dimension)of Ni90Cr10were deposited on the three-layer dielectric for different analytical studies.Ni90Cr10 pads were also deposited on insulated Si substrates for electrical reliability test and to study interdiffusion.The fabrication of Ni90Cr10pads on SS and Si is illustrated in Fig.1.Fig.1shows all the steps used to fabricate samples for pad tests,both on SS [Fig.1(a)]and on Si[Fig.1(b)].Resistance was measured between the Ni90Cr10pad and the underlying substrate(SS or chromel sputtered on Si)with an ohmmeter to detect an electrical short.Since microelectronic grade SS substrates are not available commercially,conventional grinding and polish-ing techniques were employed to obtain a smooth surface with Root-Mean-Square roughness smaller than100nm that is suitable for microfabrication,The substrate surface roughness was measured using a Zygo New View white light inter-ferometer.As mentioned earlier,the aim of this study was to investigate the behavior of Ni90Cr10thin films at elevated temperature,especially interdiffusion and potential chemical reactions.Annealing was carried out in diffusion bonding equipment capable of reaching a vacuum level on the order of ~10−3Pa and temperatures as high as1800°C.The annealing temperature curve is shown in Fig.2.Note that800°C was selected as annealing temperature,because it is within thetypical temperature range for diffusion bonding of stainless steel to both stainless steel and many other materials such as titanium alloy and copper[10,11].Resistance/sheet resistance measure-ments,X-ray Diffraction(XRD),X-ray Photoelectron Spectro-scopy(XPS)and Scanning Electron Microscopy(SEM)with Energy Dispersive Spectroscopy(EDS)were utilized to characterize the samples after heat treatment.In order to study possible phase formation after annealing due to potential chemical reaction and interdiffusion,XRD was performed on both as-deposited and annealed Ni90Cr10films using a Bruker-AXS GADDS(Hi-Star)2D X-Ray diffract-ometer Hi-star multi-wire with two-dimensional area detector and Cu X rays.During analysis,the sample surface was bombarded with energetic argon ions to sputter material away at regular intervals and the concentration of each relevant element was estimated after each sputtering cycle.A pad test was designed to investigate the electrical failure of Ni90Cr10pads if shorted to the substrate.Cross-sectional SEM,EDS line scan, and EDS mapping were performed to study interdiffusion after annealing at elevated temperatures using a LEO GEMINI1530 SEM with a Schottky-type field emission and EDS x-ray analyzer.The SEM operating voltage was set at15kV.The annealing temperature was set to800°C,which is sufficient for various practical applications,and was carried out inside a diffusion bonder because diffusion bonding is being considered as one of the most promising techniques for thin film micro/ nano sensors embedding into metals and ceramics.The thickness of chromel films was460–470nm for allstudies. Fig.1.Fabrication of Ni90Cr10(chromel)pads on(a)Stainless Steel and(b) Silicon substrates.4308 A.Datta et al./Thin Solid Films516(2008)4307–4311Very thin films (100nm or less)were avoided,as they are relatively more susceptible to oxidation than films with thickness around 500nm and greater [12,13].Although the annealing was typically done at 4×10−3Pa,oxygen could not be completely removed from the chamber.Annealing was carried out in such atmosphere to find out the effect of this residual oxygen on the surface of the Ni90Cr10films during annealing.XPS was performed using a Perkin-Elmer PHI 5400XPS spectrophotometer (Mg X-ray source)to examine the depth profile of Ni,Cr and O elements.Following an initial set of XPS survey as a baseline;each sample was sputter etched using 3kV Ar+in 5-min intervals and XPS survey taken after each etch cycle (total 12cycles).The take off angle was 45°,and the current of ion beam was at 2.5μA.The sensitivity factors used for atomic concentration estimates were 1.583for Cr 2p3,2.696for Ni2p3,and 0.711fir O 1s.3.Results and discussionsResistivity of Ni90Cr10films was measured by four point probe method before and after annealing at 800°C.The value of the resistivity of as-deposited films on sapphire substrates (~470nm thick)was 73μΩ-cm,which reduced to about 67μΩ-cm after annealing at 800°C.Resistivity of 470nm Ni90Cr10films did not change much after annealing and matched closely with the reported value [5].Sapphire substrates were used for resistivity measurement,as they were stochiometric and provide a perfect insulation material eliminating any influence from underlying layers on resistivity measurements of Ni90Cr10films.Fig.3shows an XPS depth profile for both as-deposited and annealed chromel films on SS substrates.Although as-deposited samples showed reasonable concentrations of Ni,Cr,and O near the surface,annealed sample showed negligible Ni content and increased Cr and O concentration.This suggested that the SS samples were exposed to sufficient O 2during annealing and that Cr segregated near the surface,resulting in a layer of chromium oxide which prevented more severe oxidation.XRD results,as shown in Fig.4,indicated that peaks matching closely to Ni were only identified before and after annealing.However,peaksfor as-deposited samples were shifted to a slightly lower angle.It is possible that the as-deposited specimens show reflections from Ni90Cr10alloy lattice whereas the annealed samples show pure Ni peaks.This is because of the segregation of Cr from Ni after annealing at high temperature,which is consistent with the XPS results.It was also expected that XRD would show the chromium oxide phase(s),but none was found.This absence could be attributed to the formation of only amorphous oxides very near the surface at the conditions (time and temperature)of annealing.During past experiments of fabricating sensors on polished SS substrates,it was noted that sensors or sensor material pads were occasionally electrically shorted to substrates after annealing.This could be due to two main reasons,either 1)imperfect substrate surfaces even after polishing or 2)interdiffusion of metal atoms from the adhesion layer or substrate triggered by high temperature annealing.To investi-gate this issue,a pad test experiment was designed.TheFig.4.X-ray diffraction (XRD)results of samples on stainless steel before and afterannealing.Fig.3.X-ray photoelectron spectroscopy (XPS)depth profile of samples on stainless steel before and after annealing at 800°C.Fig.2.Annealing scheme of samples in diffusion bonder.4309A.Datta et al./Thin Solid Films 516(2008)4307–4311fabrication and test scheme of chromel pads is shown in Fig.1,both on Si and polished SS substrates.Silicon served as an ideal substrate to rule out any failure due to a surface imperfection.Electrical pad tests (measurement of resistance between pad and underlying chromel layer on Si)were performed before and after annealing.No pads were found shorted in either case,indicating no significant interdiffusion that could lead to failure of the sensor layer.This confirmed the reliability of the dielectric combination indirectly.In the case of SS substrates,about 70%of pads survived the annealing process which suggested that failure was due to surface quality but not due toany interdiffusion.To obtain a better insight to the interdiffusion phenomena,cross-sectional SEM/EDS was conducted on the SS samples.The results are shown in Figs.5and 6.Note that epoxy was used to mount samples for polishing.Besides being fabricated on dielectric layers,the chromel films were also covered by the three-layer dielectric (Al 2O 3/Si x N y /Al 2O 3)from the top in order to study interdiffusion in a real sensor structure where the sensor would usually be embedded in symmetric dielectric layers.EDS mapping for as-deposited and annealed samples showed little difference.No severe interdiffusion of Cr or Fe into dielectric layers was noticed after annealing.These results are consistent with XPS and electrical pad test results previouslydiscussed.Fig.6.Cross-sectional SEM image of (a)annealed sample on stainless steel and (b)corresponding EDSmapping.Fig.5.Cross-sectional SEM image of (a)asdeposited sample on stainless steel and (b)corresponding EDS mapping.4310 A.Datta et al./Thin Solid Films 516(2008)4307–43114.ConclusionsChromel(Ni90Cr10)thin films,an important material in temperature sensing,were annealed at800°C in a diffusion bonder under vacuum.Also note that the atmosphere inside the bonder is not totally oxygen free since the vacuum was at 1.33×10−3Pa.A three-layer dielectric(Al2O3/Si x N y/Al2O3) was deposited on a very thin Cr adhesion layer that was sputtered onto a polished SS substrate.From the XPS study,it was found that Cr segregated from Ni after high temperature annealing and probably formed chromium oxide on the surface,preventing further oxidation.XRD could not detect any chromium oxide phase,only the Ni phase.As-deposited samples showed a slight shift in peak positions compared to the annealed samples. Electrical pad test on Si substrate showed that there was no short-circuit of chromel thin film pads to the bottom chromel layer after annealing.About70%of pads on SS substrates remained un-shorted in similar experiments.Pad test experiments proved that there was no failure related to interdiffusion.Cross-sectional EDS mapping was also conducted on annealed and as-deposited samples to verify the findings from electrical pad tests.An EDS study suggested that little interdiffsuion occurs in the thin film systems after annealing.In short,the present combination of dielectrics was quite reliable and Ni90Cr10films of500nm could be used at elevated temperatures up to800°C in a diffusion bonding environment.AcknowledgementsThe authors thankfully acknowledge the support from National Science Foundation(NSF).References[1]C.L.M Au,M.A.Jackson,W.A.Anderson,J.Electron.Mater.16(1987)301.[2]G.Nocerino,K.E.Singer,J.Vac.Sci.Technol.16(1979)147.[3]S.Hofmann,A.Zalar,Thin Solid Films39(1976)219.[4]ssak,K.Hieber,Thin Solid Films17(1973)105.[5]H.Choi,A.Datta,X.Cheng,X.Li,J.Microelectromech.Syst.15(2006)322.[6]X.Zhang,H.Choi,A.Datta,X.Li,J.Micromech.Microeng.16(2006)900.[7]N.M.Phuong,D.J.Kim,B.D.Kang,S.G.Yoon,J.Electrochem.Soc.153(2006)G660.[8]R.Kriukiene,S.Tamulevicius,Mater.Sci.(Lithuania)10(2004)136.[9]A.Datta,H.Choi,X.Li,J.Electrochem.Soc.153(2006)H89.[10]N.Orhan,T.I.Kha,M.Eroglu,Scripta.Mater.45(2001)441.[11]O.Yilmaz,Mater.Sci.Technol.17(2001)989.[12]M.Kamaratos,D.Vlachos,S.D.Foulias,Ch.Argirusis,Surf.Rev.Lett.11(2004)419.[13]L.Zhao,G.Shen,G.Gao,C.Xu,J.Du,D.Zou,J.Chen,in:M.Zhang,K.N.Tu(Eds.),Proc.5th International Conference of Solid-State and Integrated Circuit Technology,Beijing,China,October21–23,1998, p.123.4311A.Datta et al./Thin Solid Films516(2008)4307–4311。
CuInS_2和CuIn_(1-x)Ga_xS_2光电材料的制备及其能带计算
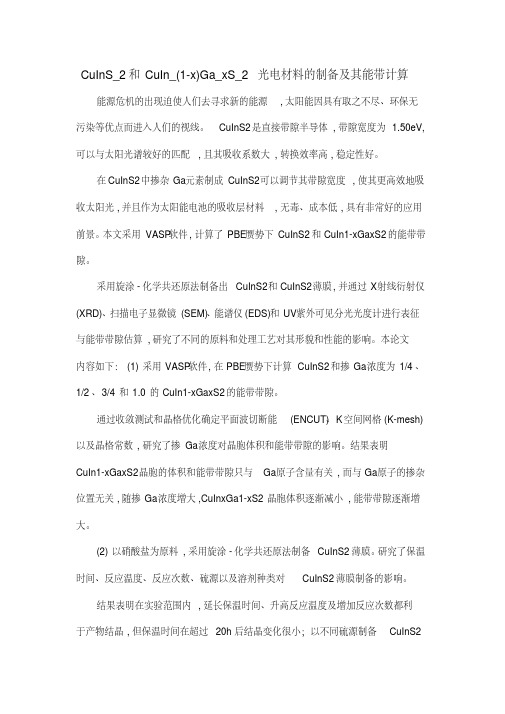
CuInS_2和CuIn_(1-x)Ga_xS_2光电材料的制备及其能带计算能源危机的出现迫使人们去寻求新的能源,太阳能因具有取之不尽、环保无污染等优点而进入人们的视线。
CuInS2是直接带隙半导体,带隙宽度为 1.50eV,可以与太阳光谱较好的匹配,且其吸收系数大,转换效率高,稳定性好。
在CuInS2中掺杂Ga元素制成CuInS2可以调节其带隙宽度,使其更高效地吸收太阳光,并且作为太阳能电池的吸收层材料,无毒、成本低,具有非常好的应用前景。
本文采用VASP软件,计算了PBE赝势下CuInS2和CuIn1-xGaxS2的能带带隙。
采用旋涂-化学共还原法制备出CuInS2和CuInS2薄膜,并通过X射线衍射仪(XRD)、扫描电子显微镜(SEM)、能谱仪(EDS)和UV紫外可见分光光度计进行表征与能带带隙估算,研究了不同的原料和处理工艺对其形貌和性能的影响。
本论文内容如下:(1)采用VASP软件,在PBE赝势下计算CuInS2和掺Ga浓度为1/4、1/2、3/4和1.0的CuIn1-xGaxS2的能带带隙。
通过收敛测试和晶格优化确定平面波切断能(ENCUT)、K空间网格(K-mesh)以及晶格常数,研究了掺Ga浓度对晶胞体积和能带带隙的影响。
结果表明CuIn1-xGaxS2晶胞的体积和能带带隙只与Ga原子含量有关,而与Ga原子的掺杂位置无关,随掺Ga浓度增大,CuInxGa1-xS2晶胞体积逐渐减小,能带带隙逐渐增大。
(2)以硝酸盐为原料,采用旋涂-化学共还原法制备CuInS2薄膜。
研究了保温时间、反应温度、反应次数、硫源以及溶剂种类对CuInS2薄膜制备的影响。
结果表明在实验范围内,延长保温时间、升高反应温度及增加反应次数都利于产物结晶,但保温时间在超过20h后结晶变化很小;以不同硫源制备CuInS2薄膜,硫脲作为硫源时产物衍射峰最好,在(112)晶面择优生长,结晶度最好,是最合适的硫源;选用不同溶剂制备CuInS2薄膜,无水乙醇为溶剂时产物衍射峰和结晶度明显好于乙醇胺和乙二胺,是最合适的溶剂。
- 1、下载文档前请自行甄别文档内容的完整性,平台不提供额外的编辑、内容补充、找答案等附加服务。
- 2、"仅部分预览"的文档,不可在线预览部分如存在完整性等问题,可反馈申请退款(可完整预览的文档不适用该条件!)。
- 3、如文档侵犯您的权益,请联系客服反馈,我们会尽快为您处理(人工客服工作时间:9:00-18:30)。
Ultrathin Topological Insulator Bi2Se3 Nanoribbons Exfoliated by Atomic Force MicroscopySeung Sae Hong,†Worasom Kundhikanjana,†Judy J.Cha,‡Keji Lai,†Desheng Kong,‡Stefan Meister,‡Michael A.Kelly,‡Zhi-Xun Shen,†and Yi Cui*,‡†Department of Applied Physics and‡Department of Materials Science and Engineering,Stanford University, Stanford,California94305ABSTRACT Ultrathin topological insulator nanostructures,in which coupling between top and bottom surface states takes place,are of great intellectual and practical importance.Due to the weak van der Waals interaction between adjacent quintuple layers(QLs), the layered bismuth selenide(Bi2Se3),a single Dirac-cone topological insulator with a large bulk gap,can be exfoliated down to a few QLs.In this paper,we report thefirst controlled mechanical exfoliation of Bi2Se3nanoribbons(>50QLs)by an atomic force microscope (AFM)tip down to a single QL.Microwave impedance microscopy is employed to map out the local conductivity of such ultrathin nanoribbons,showing drastic difference in sheet resistance between1-2QLs and4-5QLs.Transport measurement carried out on an exfoliated(e5QLs)Bi2Se3device shows nonmetallic temperature dependence of resistance,in sharp contrast to the metallic behavior seen in thick(>50QLs)ribbons.These AFM-exfoliated thin nanoribbons afford interesting candidates for studying the transition from quantum spin Hall surface to edge states.KEYWORDS Topological insulator,bismuth selenide,nanoribbon,mechanical exfoliation,atomic force microscopyT he metallic surface states of3D topological insulators1-7 are protected from disorder effects such as crystaldefects and nonmagnetic impurities,promising real-ization of dissipationless electron transport in the absence of high magneticfields.8After the initial discovery of the2D quantum spin Hall effect(QSHE)in HgTe quantum wells,7,9 three binary compounds,Bi2Se3,Bi2Te3,and Sb2Te3,were predicted and later confirmed by angle-resolved photoemis-sion spectroscopy(ARPES)as3D topological insulators.10-13 In particular,Bi2Se3has been studied due to the relatively large bulk band gap(∼0.3eV)and the simple band structure near the Dirac point.12-17Many exotic physical phenomena are predicted to emerge in low dimensional nanostructures of Bi2Se3.18,19For example,ultrathin Bi2Se3down to a few (e5)nanometers is expected to exhibit topologically non-trivial edge states,which serves as a new platform for the 2D QSHE.18In addition,tuning of the chemical potential becomes easier than thick Bi2Se3due to the suppression of bulk contribution.Fortunately,such ultrathin Bi2Se3can be naturally obtained due to its layered rhombohedral crystal structure;two Bi and three Se atomic sheets are covalently bonded to form one quintuple layer(QL,∼1nm thick) (Figure1b),where adjacent QLs are coupled by relatively weak van der Waals interaction.Such anisotropic bonding structure implies that similar to the case of graphene20low dimensional crystals of Bi2Se3can be generated by mechanical exfoliation,which has been achieved by several groups.21-23 However,the obtainedflakes are usually irregular in shape and the yield of obtaining ultrathinflakes is low:the typical reportedflakes(∼10nm)are still thick compared with the*To whom correspondence should be addressed,yicui@. Received for review:05/27/2010Published on Web:07/12/2010FIGURE1.(a)Schematic of AFM exfoliation of layered structure nanomaterial-Bi2Se3nanoribbon.Horizontal tip force(y-direction) is applied on the side of a nanoribbon to break the in-plane covalent bonding.(b)Crystal structure of Bi2Se3.One quintuple layer(QL) consists offive atomic layers of Bi and Se(Se-Bi-Se-Bi-Se).(c)The horizontal tip force is from torsional displacement( )of the tip. When the tip is scanning across a nanoribbon without z-directional feedback,the whole cantilever is twisted so that the tip can go over step edge d.The shear stress on the AFM cantilever generates a torque on the tip end,which applies the horizontal tip force to thenanoribbon.2D limit where strong coupling between top and bottom surfaces occurs.Therefore,thinning Bi 2Se 3in a controlled manner is desirable.Here we demonstrate a new method to exfoliate Bi 2Se 3by dragging an atomic force microscopy (AFM)tip 24horizontally across the Bi 2Se 3nanoribbons.14,25-28If the horizontal force applied by the AFM tip is large enough to break the Bi -Se bonds (Figure 1a),the upper portion of the ribbon will be scratched away.By controlling the lateral force and vertical position of the tip,it is possible to remove most of upper QLs and have intact ultrathin Bi 2Se 3at the bottom.Figure 2illustrates the AFM exfoliation procedure of a Bi 2Se 3nanoribbon.Bi 2Se 3nanoribbons are initially synthe-sized by vapor -liquid -solid (VLS)method using Au catalyst particles on silicon substrate.25The thicknesses of Bi 2Se 3nanoribbons are 25-100nm.They are transferred to an oxidized silicon (Si/300nm SiO 2)substrate for exfoliation.In order to increase surface friction,the substrate is pre-treated with organic polymer (i.e.,poly(methyl methacry-late),PMMA)and annealed (200-400°C)in an argon atmosphere after the nanoribbon transfer.A commercial AFM tip made of silicon is used in contact mode,to exfoliate Bi 2Se 3nanoribbon.The AFM tip approaches a Bi 2Se 3nano-ribbon in the horizontal direction,and scans across the nano-ribbons (50-100nm thick)at a constant height (Figure 2a).When the tip apex is lower than the top surface of the Bi 2Se 3nanoribbon,horizontal movement applies force and breaks the nanoribbon,leaving some residual intact layers under-neath the broken part (Figure 2b -e).By repeating this scan on a single nanoribbon multiple times,we are able to thin down thick nanoribbons (∼100nm)to a few QLs,while maintaining the original lateral length of about several micrometers.After the AFM exfoliation,the topography of the nano-ribbons is characterized by the usual AFM function.The observed thicknesses vary from ribbons to ribbons,but usually between 1and 15nm.A few examples of ultrathin Bi 2Se 3nanoribbons are shown in Figure 3.A majority of the exfoliated ribbons have uniform thickness (Figure 3a -d),but sometimes we also observe discrete thickness steps within a single ribbon (Figure 3e,f).We note that the thickness of thin nanoribbons changes by multiples of the height of one QL (∼1nm),which is expected from the weak bonding between two adjacent QLs.The AFM exfoliation mechanism can be understood by comparing the horizontal force due to torsion of the AFM cantilever with the bonding strength of Bi 2Se 3(Figure 1c).When an AFM tip scans through a nanoribbon while main-taining its height,it is tilted (with respect to the x axis)to go over the sidewall.The torsional rotation of the cantilever produces shear stress,which is linearly proportional to the rotation angle and applies torque on the side of the nanoribbon.The maximum force F max applicable by the AFM tip can be estimated from the maximum rotation max due to the nanoribbon thickness d ,as the equation below 29FIGURE 2.(a,b)While the tip force breaks covalent bonding of upper layers,weak interlayer bonding,van der Waals bonding,is also broken in the middle of the nanoribbon.As a result,several bottom layers remained intact while the AFM tip breaks most of the Bi 2Se 3layers.(c -e)AFM topographic image of a Bi 2Se 3nanoribbon (d ∼50nm)before breaking (c)and after the breaking (d),and the zoom-in image (e).The bottom layers are not broken apart and maintain the same thickness (∼2nm).All scale bars indicate 500nm.FIGURE 3.Topographic images and height profiles of very thin Bi 2Se 3nanoribbons exfoliated by AFM,corresponding to 1QL (a,b),3QLs (c,d),and 1QL/4QLs (e,f).Red lines indicate the location of the height profiles.where J is the cantilever’s polar moment of inertia,G theshear modulus,L the cantilever length,and R the tip height.In the case of a typical AFM tip made of Si(G)79.9GPa)on100nm thick nanoribbon,the maximum force is about3.4mN.For a typical nanoribbon(100nm in thickness and1µm in width),the estimated covalent bonding force isabout1-2mN,which is comparable order to the maximumtip force(see Supporting Information).In other words,fornanostructures of small cross-sectional area,the AFM tip canapply enough force to break them.We note that the verticaltip force(∼10nN)does not contribute to the exfoliation ofBi2Se3nanoribbons;in fact,this force is usually kept smallto minimize surface friction and avoid potential damage tothe surface of ultrathin nanoribbon.The electrical properties of the exfoliated Bi2Se3nanorib-bons are characterized by both transport and scanningmicrowave impedance microscopy(MIM)studies.Whiletransport measurement provides quantitative informationof individual nanoribbons by lithographically patterning dcelectrodes,MIM is much more efficient in obtaining semi-quantitative local conductivity on a large number of pristinematerials.30,31The setup is implemented on a commercialAFM so that the topographic and electrical information canbe obtained simultaneously.In a MIM measurement(Figure4a),the real(MIM-R)and imaginary(MIM-C)parts of the localsample impedance at1GHz are detected to form near-fieldmicrowave images.The MIM signals can be understood bymodeling the impedance response as a function of the sheetresistance(R s)of the nanoribbon(Figure4b).For a highlyconducting ribbon with R s<10kΩ/square,a strong MIM-C signal and zero MIM-R signal are expected,taking the Si/SiO2substrate as a reference.For a highly resistive ribbon withR s>10MΩ/square,on the other hand,both MIM-C and MIM-R signals are negligibly small.Exfoliated Bi2Se3nanoribbons offive different thicknesses(1,2,4,5,6QLs)are scanned by MIM at room temperature.Panels c-f of Figure4show the MIM and AFM images and linecuts from a particular Bi2Se3nanoribbon with different thick-nesses.The number of layers(1,4,5QLs)can be identified inthe topographic image and the corresponding line cut(Figure4c,e).The simultaneously taken MIM-C image in Figure4dshows qualitative difference from the AFM data.On one hand,the part of the nanoribbon with4and5QLs shows strong anduniform MIM-C signal and zero MIM-R(line cut,Figure4f)response,indicating low R s<10kΩ/square,consistent with the transport data on the same ribbon(will be discussed later).Onthe other hand,the part of the nanoribbon with only1QLshows little signal in both MIM-C and MIM-R channels,implyingvery high local R s>10MΩ/square.Indeed,we have studied multiple Bi2Se3nanoribbons with1or2QLs and measurednegligible conductivity by MIM.Recent theoretical calculations and ARPES measurements have addressed the3D to2D crossover of ultrathin topologi-cal insulators.17,18For Bi2Se3down to1and2QLs,large energy gaps open up in both the bulk and the surface states, whereas for3QLs and above the surface gap starts toF max)JGLRmax)JGLR d R(1)FIGURE4.(a)Schematic of the microwave impedance microscopy.TheBi2Se3sample is on Si/300nm SiO2substrate.A Pt tip scans on thesample surface and a microwave signal(f)1GHz)is guided to the tipapex.The impedance responses are recorded as capacitive signal(MIM-C)and resistive signal(MIM-R)after phase-sensitive demodulation.(b)Simulated MIM signals with respect to the Si/SiO2substrate saturatesfor both low(R s<10kΩ/square)and high R s>10MΩ/square)sheetresistance and decreases monotonically in between,in which MIM-Rsignal is detected.The measured R s is from transport data of the samedevice.(c)Topographic image of an exfoliated nanoribbon with threedifferent thicknesses(1QL,4QLs,5QLs)in different regions.(d)MIM-Cimage of the nanoribbon in(c).Strong and uniform MIM-C signals areseen in the4and5QLs regions,consistent with the low resistivity.Onthe other hand,1QL(edge of the ribbon indicated by broken line)areashows little MIM-C signal,implying high local resistivity.Scale bars in(c,d)are500nm and green lines correspond to the profiles in(e,f).(e)A line cut from(c),showing1,4,and5QLs on the substrate.(f)Microwave signals at the same line.Note that the MIM-C signal on the1QL section is caused by parasitic capacitance from the nearby metallic4,5QLs.The negligible MIM-R signal throughout the ribbon confirmsthe high conductivity in4,5QLs and low conductivity in1QL Bi2Se3.diminish.Therefore,if the chemical potential happens to fall inside the gap for our 1and 2QLs of Bi 2Se 3,R s could be quite large even at the room temperature,which agrees with the MIM results.However,there exist other practical issues re-sponsible for the observed high R s .One possibility may be that the 1or 2QL nanoribbons are significantly oxidized in the ambient condition.From our experience,thin Bi 2Se 3nanorib-bons appear very sensitive to moisture and oxygen in the surrounding environment.Further experiments are needed to distinguish the causes for the observed insulating behavior of 1and 2QLs of Bi 2Se 3.The low R s in the 4-6QLs samples can be explained by the small surface gaps and the selenium (Se)vacancies,which work as effective n-type dopants in Bi 2Se 3crystals.It is likely that the chemical potential is actually located in the bulk conduction band.11,13For the 4-6QLs,even if the outmost 1-2QLs are affected by the environment and become insulating,there remain enough Bi 2Se 3QLs in the middle that contribute to the small R s .dc transport measurements are also performed on ul-trathin nanoribbons.A device with four-probe geometry is fabricated from an AFM-exfoliated Bi 2Se 3nanoribbon with 5QLs by standard electron beam lithography and thermal evaporation of Cr/Au contacts (Figure 5b).At room temper-ature,the measured R s (2.8k Ω/square)is consistent with the MIM results in the previous section.The transport data of another device fabricated on an as-grown nanoribbon (82nm thick)are also shown in Figure 5a for comparison.We note that the room temperature conductivity (σ)1/R s t )difference between this thick ribbon (3.7×104S/m)and the exfoliated device described above (7.2×104S/m)falls well within the variation from same batch of VLS growth.Interestingly,these two devices behave differently in the temperature dependence.The resistance of as-grown nan-oribbon decreases as temperature goes down to 2K (R s )127Ω/square),which is typically observed in most of Bi 2Se 3nanoribbon devices (Figure 5c,d).14This metallic behavior of Bi 2Se 3nanoribbons is expected for heavily doped semi-conductors as discussed earlier.On the other hand,the resistance of the 5QLs Bi 2Se 3nanoribbon increases as temperature goes down.Unlike the usual band insulator,however,its resistance does not increase exponentially at low temperature but saturates toward a finite number R s )4.9k Ω/square at our lowest temperature of 2K (Figure 5c,d).Such nonmetallic behavior is not reported in exfoliated Bi 2Se 3before.Since the outmost 1-2QLs are shown to be insulating due to surface chemistry in the ambient environ-ment,it is possible that the chemical potential in the remain-ing intact layers gets close to bulk and surface gaps.The residual conductance is then due to the edge conducting channels only.Further experiments ruling out trivial extrin-sic effects are needed to support this hypothesis.In summary,for the first time,we demonstrate the mechanical exfoliation of layered nanoribbons by AFM,which is very effective and powerful technique to generate ultrathin layered structure.In the example of Bi 2Se 3nano-ribbons,exfoliated layers from 1QL (∼1nm)to multiple QLs are acquired and confirmed by AFM scans on multiple samples.Microwave impedance microscopy reveals the local electronic property of pristine Bi 2Se 3layers and shows that exfoliated nanoribbons thicker than 3QLs are highly conductive,which is consistent with the room temperature transport measurement.Nonmetallic temperature depen-dence of resistance,distinct from that of as-grown Bi 2Se 3nanoribbons,is observed in the ultrathin Bi 2Se 3device,leaving an interesting subject for future study.Acknowledgment.Y.C.acknowledges the support from the Keck Foundation.This work is also made possible by the King Abdullah University of Science and Technology (KAUST)Investigator Award (No.KUS-l1-001-12)and KAUST GRP Fellowship (No.KUS-F1-033-02),NSF Grant DMR-0906027,and Center of Probing the Nanoscale,Stanford University (NSF Grant PHY-0425897).Supporting Information Available.Sample preparation method,characterization methods,and microwave/trans-port measurement details,and additional force calculations and data.This material is available free of charge via the Internet at .REFERENCES AND NOTES(1)Qi,X.;Zhang,S.Phys.Today 2010,63,33–38.(2)Moore,J.E.Nature 2010,464,194–198.(3)Fu,L.;Kane,C.Phys.Rev.B 2007,76,4045302.(4)Kane,C.;Mele,E.Phys.Rev.Lett.2005,95,146802.FIGURE 5.Electrical transport experiments on exfoliated nanoribbon and as-grown nanoribbon.(a)Scanning electron microscopy (SEM)image of the as-grown nanoribbon device with multiple contacts and a front gate (not discussed here).Inset indicates the nanoribbon thickness (82nm).(b)SEM image of the exfoliated nanoribbon device.Since very thin Bi 2Se 3is barely distinguishable in SEM,the AFM image is overlaid to show the device geometry.Several broken segment of the original nanoribbon from exfoliation are seen near the electrodes.A line cut in the inset shows the thickness of this nanoribbon.Both scale bars in (a)and (b)are 5µm.(c)Resistance R (four-point,log scale)vs temperature T of the two devices in (a)and (b).R deceases as decreasing T for the as-grown nanoribbon (blue)but increases for the exfoliated nanoribbon (red).(d)R (log scale)vs 1000/T .The as-grown nanoribbon shows metallic temper-ature dependence,while the exfoliated nanoribbon’s resistance increases during cool down and saturates near 2K.(5)Bernevig,B.;Zhang,S.Phys.Rev.Lett.2006,96,106802.(6)Zhang,H.;Liu,C.;Qi,X.;Dai,X.;Fang,Z.;Zhang,S.Nat.Phys.2009,5,438–442.(7)Bernevig,B.A.;Hughes,T.L.;Zhang,S.Science2006,314,1757–1761.(8)Kane,C.L.;Mele,E.J.Science2006,314,1692–1693.(9)Konig,M.;Wiedmann,S.;Brune,C.;Roth,A.;Buhmann,H.;Molenkamp,L.W.;Qi,X.;Zhang,S.Science2007,318,766–770.(10)Hsieh,D.;Qian,D.;Wray,L.;Xia,Y.;Hor,Y.S.;Cava,R.J.;Hasan,M.Z.Nature2008,452,970–974.(11)Chen,Y.L.;Analytis,J.G.;Chu,J.;Liu,Z.K.;Mo,S.;Qi,X.L.;Zhang,H.J.;Lu,D.H.;Dai,X.;Fang,Z.;Zhang,S.C.;Fisher,I.R.;Hussain,Z.;Shen,Z.Science2009,325,178–181.(12)Xia,Y.;Qian,D.;Hsieh,D.;Wray,L.;Pal,A.;Lin,H.;Bansil,A.;Grauer,D.;Hor,Y.S.;Cava,R.J.;Hasan,M.Z.Nat.Phys.2009, 5,398–402.(13)Hsieh,D.;Xia,Y.;Qian,D.;Wray,L.;Dil,J.H.;Meier,F.;Osterwalder,J.;Patthey,L.;Checkelsky,J.G.;Ong,N.P.;Fedorov,A.V.;Lin,H.;Bansil,A.;Grauer,D.;Hor,Y.S.;Cava,R.J.;Hasan,M.Z.Nature2009,460,1101–1105.(14)Peng,H.;Lai,K.;Kong,D.;Meister,S.;Chen,Y.;Qi,X.;Zhang,S.;Shen,Z.;Cui,Y.Nat.Mater.2010,9,225–229.(15)Checkelsky,J.;Hor,Y.;Liu,M.;Qu,D.;Cava,R.;Ong,N.Phys.Rev.Lett.2009,103,246601.(16)Analytis,J.G.;McDonald,R.D.;Riggs,S.C.;Chu,J.-H.;Boebinger,G.S.;Fisher,I.R.Preprinted at /abs/1003.1713.(17)Zhang,Y.;He,K.;Chang,C.;Song,C.;Wang,L.;Chen,X.;Jia,J.;Fang,Z.;Dai,X.;Shan,W.;Shen,S.;Niu,Q.;Qi,X.;Zhang,S.;Ma,X.;Xue,Q.Preprinted at /abs/0911.3706.(18)Liu,C.;Zhang,H.;Yan,B.;Qi,X.;Frauenheim,T.;Dai,X.;Fang,Z.;Zhang,S.Phys.Rev.B2010,81,No.041307.(19)Seradjeh,B.;Moore,J.E.;Franz,M.Phys.Rev.Lett.2009,103,No.066402.(20)Novoselov,K.S.;Jiang,D.;Schedin,F.;Booth,T.J.;Khotkevich,V.V.;Morozov,S.V.;Geim,A.K.Proc.Natl.Acad.Sci.U.S.A.2005, 102,10451–10453.(21)Checkelsky,J.G.;Hor,Y.S.;Cava,R.J.;Ong,N.P.Preprinted at/abs/1003.3883.(22)Teweldebrhan,D.;Goyal,V.;Balandin,A.A.Nano Lett.2010,10,1209–1218.(23)Steinberg,H.;Gardner,D.R.;Lee,Y.S.;Jarillo-Herrero,P.Preprinted at /abs/1003.3137.(24)Kim,Y.;Lieber,C.M.Science1992,257,375–377.(25)Kong,D.;Randel,J.C.;Peng,H.;Cha,J.J.;Meister,S.;Lai,K.;Chen,Y.;Shen,Z.;Manoharan,H.C.;Cui,Y.Nano Lett.2010, 10,329–333.(26)Cha,J.J.;Williams,J.R.;Kong,D.;Meister,S.;Peng,H.;Bestwick,A.J.;Gallagher,P.;Goldhaber-Gordon,D.;Cui,Y.Nano Lett.2010,10,1076–1081.(27)Kong,D.;Dang,W.;Cha,J.J.;Li,H.;Meister,S.;Peng,H.;Liu,Z.;Cui,Y.Preprinted at /abs/1004.1767. (28)Tang,H.;Liang,D.;Qiu,R.L.J.;Gao,X.P.A.Preprinted at http:///abs/1003.6099.(29)Ugural,A.C.;Fenster,S.K.Advanced Strength and AppliedElasticity,4th ed.;Prentice Hall:Englewood Cliffs,NJ,2003. (30)Lai,K.;Peng,H.;Kundhikanjana,W.;Schoen,D.T.;Xie,C.;Meister,S.;Cui,Y.;Kelly,M.A.;Shen,Z.Nano Lett.2009,9, 1265–1269.(31)Lai,K.;Kundhikanjana,W.;Kelly,M.;Shen,Z.X.Rev.Sci.Instrum.2008,79,No.063703.。