多传感器检测系统信号处理研究外文翻译
sensors and actuators reports的缩写形式
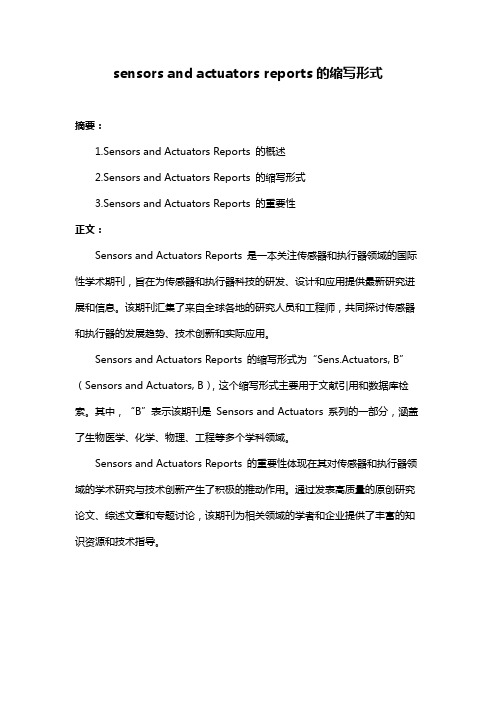
sensors and actuators reports的缩写形式
摘要:
1.Sensors and Actuators Reports 的概述
2.Sensors and Actuators Reports 的缩写形式
3.Sensors and Actuators Reports 的重要性
正文:
Sensors and Actuators Reports 是一本关注传感器和执行器领域的国际性学术期刊,旨在为传感器和执行器科技的研发、设计和应用提供最新研究进展和信息。
该期刊汇集了来自全球各地的研究人员和工程师,共同探讨传感器和执行器的发展趋势、技术创新和实际应用。
Sensors and Actuators Reports 的缩写形式为“Sens.Actuators, B”(Sensors and Actuators, B),这个缩写形式主要用于文献引用和数据库检索。
其中,“B”表示该期刊是Sensors and Actuators 系列的一部分,涵盖了生物医学、化学、物理、工程等多个学科领域。
Sensors and Actuators Reports 的重要性体现在其对传感器和执行器领域的学术研究与技术创新产生了积极的推动作用。
通过发表高质量的原创研究论文、综述文章和专题讨论,该期刊为相关领域的学者和企业提供了丰富的知识资源和技术指导。
传感器技术论文中英文对照资料外文翻译文献
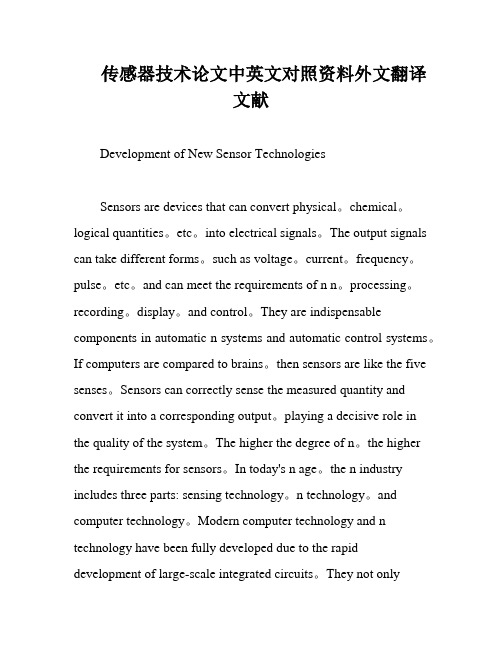
传感器技术论文中英文对照资料外文翻译文献Development of New Sensor TechnologiesSensors are devices that can convert physical。
chemical。
logical quantities。
etc。
into electrical signals。
The output signals can take different forms。
such as voltage。
current。
frequency。
pulse。
etc。
and can meet the requirements of n n。
processing。
recording。
display。
and control。
They are indispensable components in automatic n systems and automatic control systems。
If computers are compared to brains。
then sensors are like the five senses。
Sensors can correctly sense the measured quantity and convert it into a corresponding output。
playing a decisive role in the quality of the system。
The higher the degree of n。
the higher the requirements for sensors。
In today's n age。
the n industry includes three parts: sensing technology。
n technology。
and computer technology。
化学实验方法外文文献翻译、中英文翻译、外文翻译
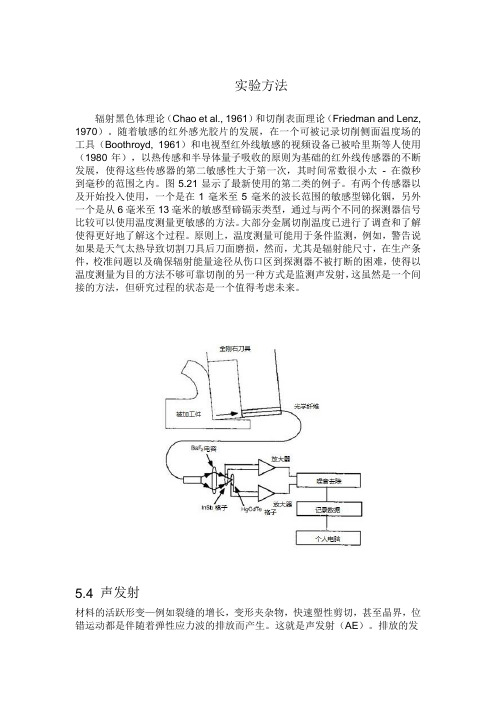
实验方法辐射黑色体理论(Chao et al., 1961)和切削表面理论(Friedman and Lenz, 1970)。
随着敏感的红外感光胶片的发展,在一个可被记录切削侧面温度场的工具(Boothroyd, 1961)和电视型红外线敏感的视频设备已被哈里斯等人使用(1980年),以热传感和半导体量子吸收的原则为基础的红外线传感器的不断发展,使得这些传感器的第二敏感性大于第一次,其时间常数很小太- 在微秒到毫秒的范围之内。
图5.21显示了最新使用的第二类的例子。
有两个传感器以及开始投入使用,一个是在1毫米至5毫米的波长范围的敏感型锑化铟,另外一个是从6毫米至13毫米的敏感型碲镉汞类型,通过与两个不同的探测器信号比较可以使用温度测量更敏感的方法。
大部分金属切削温度已进行了调查和了解使得更好地了解这个过程。
原则上,温度测量可能用于条件监测,例如,警告说如果是天气太热导致切割刀具后刀面磨损,然而,尤其是辐射能尺寸,在生产条件,校准问题以及确保辐射能量途径从伤口区到探测器不被打断的困难,使得以温度测量为目的方法不够可靠切削的另一种方式是监测声发射,这虽然是一个间接的方法,但研究过程的状态是一个值得考虑未来。
5.4 声发射材料的活跃形变—例如裂缝的增长,变形夹杂物,快速塑性剪切,甚至晶界,位错运动都是伴随着弹性应力波的排放而产生。
这就是声发射(AE)。
排放的发生在一个很宽的频率范围内,但通常是从10万赫到1兆赫。
虽然波幅度很小,但是他们可以被检测到,通过强烈的压电材料如钛酸钡或压电陶瓷传感器制造从,(Pb(Zr x Ti1–x)O3; x = 0.5 to 0.6)。
图5.22显示了传感器的结构。
声波传送到压力传感器造成直接的压力E(△L/L),其中E是传感器的杨氏模量,L 是它的长度,△L是它的长度变化。
应力产生电场T = g33E(△L/L)(5.7a)g33是传感器材料的压电应力系数。
传感器两端的电压是TL,然后V= g33E△L(5.7b)g33和E的典型值分别是24.4 × 10-3Vm/ N和58.5GPa,以检测电压高达0.01毫伏,这是可能的。
外文翻译译文模板
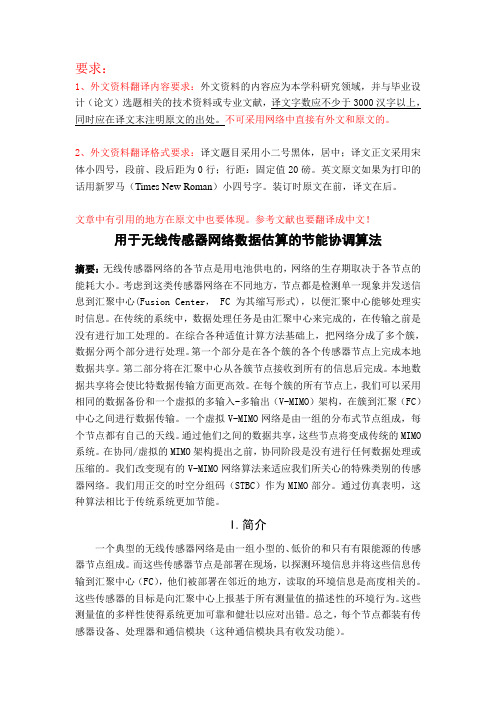
要求:1、外文资料翻译内容要求:外文资料的内容应为本学科研究领域,并与毕业设计(论文)选题相关的技术资料或专业文献,译文字数应不少于3000汉字以上,同时应在译文末注明原文的出处。
不可采用网络中直接有外文和原文的。
2、外文资料翻译格式要求:译文题目采用小二号黑体,居中;译文正文采用宋体小四号,段前、段后距为0行;行距:固定值20磅。
英文原文如果为打印的话用新罗马(Times New Roman)小四号字。
装订时原文在前,译文在后。
文章中有引用的地方在原文中也要体现。
参考文献也要翻译成中文!用于无线传感器网络数据估算的节能协调算法摘要:无线传感器网络的各节点是用电池供电的,网络的生存期取决于各节点的能耗大小。
考虑到这类传感器网络在不同地方,节点都是检测单一现象并发送信息到汇聚中心(Fusion Center, FC为其缩写形式),以便汇聚中心能够处理实时信息。
在传统的系统中,数据处理任务是由汇聚中心来完成的,在传输之前是没有进行加工处理的。
在综合各种适值计算方法基础上,把网络分成了多个簇,数据分两个部分进行处理。
第一个部分是在各个簇的各个传感器节点上完成本地数据共享。
第二部分将在汇聚中心从各簇节点接收到所有的信息后完成。
本地数据共享将会使比特数据传输方面更高效。
在每个簇的所有节点上,我们可以采用相同的数据备份和一个虚拟的多输入-多输出(V-MIMO)架构,在簇到汇聚(FC)中心之间进行数据传输。
一个虚拟V-MIMO网络是由一组的分布式节点组成,每个节点都有自己的天线。
通过他们之间的数据共享,这些节点将变成传统的MIMO 系统。
在协同/虚拟的MIMO架构提出之前,协同阶段是没有进行任何数据处理或压缩的。
我们改变现有的V-MIMO网络算法来适应我们所关心的特殊类别的传感器网络。
我们用正交的时空分组码(STBC)作为MIMO部分。
通过仿真表明,这种算法相比于传统系统更加节能。
I.简介一个典型的无线传感器网络是由一组小型的、低价的和只有有限能源的传感器节点组成。
传感器外文翻译---传感器的基础知识
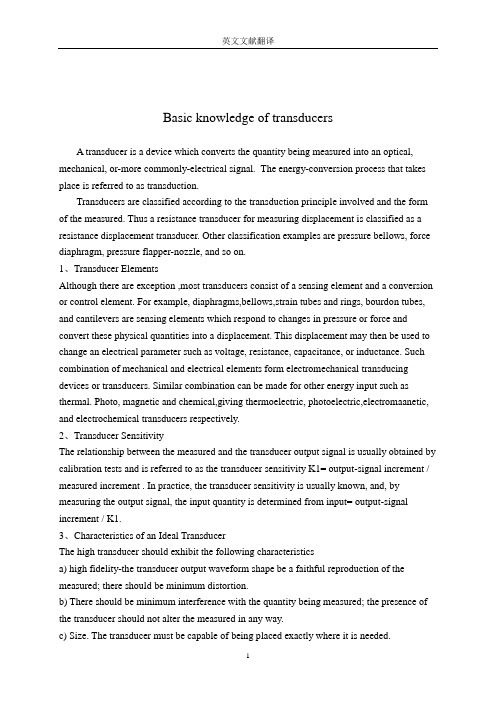
Basic knowledge of transducersA transducer is a device which converts the quantity being measured into an optical, mechanical, or-more commonly-electrical signal. The energy-conversion process that takes place is referred to as transduction.Transducers are classified according to the transduction principle involved and the form of the measured. Thus a resistance transducer for measuring displacement is classified as a resistance displacement transducer. Other classification examples are pressure bellows, force diaphragm, pressure flapper-nozzle, and so on.1、Transducer ElementsAlthough there are exception ,most transducers consist of a sensing element and a conversion or control element. For example, diaphragms,bellows,strain tubes and rings, bourdon tubes, and cantilevers are sensing elements which respond to changes in pressure or force and convert these physical quantities into a displacement. This displacement may then be used to change an electrical parameter such as voltage, resistance, capacitance, or inductance. Such combination of mechanical and electrical elements form electromechanical transducing devices or transducers. Similar combination can be made for other energy input such as thermal. Photo, magnetic and chemical,giving thermoelectric, photoelectric,electromaanetic, and electrochemical transducers respectively.2、Transducer SensitivityThe relationship between the measured and the transducer output signal is usually obtained by calibration tests and is referred to as the transducer sensitivity K1= output-signal increment / measured increment . In practice, the transducer sensitivity is usually known, and, by measuring the output signal, the input quantity is determined from input= output-signal increment / K1.3、Characteristics of an Ideal TransducerThe high transducer should exhibit the following characteristicsa) high fidelity-the transducer output waveform shape be a faithful reproduction of the measured; there should be minimum distortion.b) There should be minimum interference with the quantity being measured; the presence of the transducer should not alter the measured in any way.c) Size. The transducer must be capable of being placed exactly where it is needed.d) There should be a linear relationship between the measured and the transducer signal.e) The transducer should have minimum sensitivity to external effects, pressure transducers,for example,are often subjected to external effects such vibration and temperature.f) The natural frequency of the transducer should be well separated from the frequency and harmonics of the measurand.4、Electrical TransducersElectrical transducers exhibit many of the ideal characteristics. In addition they offer high sensitivity as well as promoting the possible of remote indication or mesdurement. Electrical transducers can be divided into two distinct groups:a) variable-control-parameter types,which include:i)resistanceii) capacitanceiii) inductanceiv) mutual-inductance typesThese transducers all rely on external excitation voltage for their operation.b) self-generating types,which includei) electromagneticii)thermoelectriciii)photoemissiveiv)piezo-electric typesThese all themselves produce an output voltage in response to the measurand input and their effects are reversible. For example, a piezo-electric transducer normally produces an output voltage in response to the deformation of a crystalline material; however, if an alternating voltage is applied across the material, the transducer exhibits the reversible effect by deforming or vibrating at the frequency of the alternating voltage.5、Resistance TransducersResistance transducers may be divided into two groups, as follows:i) Those which experience a large resistance change, measured by using potential-divider methods. Potentiometers are in this group.ii)Those which experience a small resistance change, measured by bridge-circuit methods. Examples of this group include strain gauges and resistance thermometers.5.1 PotentiometersA linear wire-wound potentiometer consists of a number of turns resistance wire wound around a non-conducting former, together with a wiping contact which travels over the barwires. The construction principles are shown in figure which indicate that the wiperdisplacement can be rotary, translational, or a combination of both to give a helical-type motion. The excitation voltage may be either a.c. or d.c. and the output voltage is proportional to the input motion, provided the measuring device has a resistance which is much greater than the potentiometer resistance.Such potentiometers suffer from the linked problem of resolution and electrical noise. Resolution is defined as the smallest detectable change in input and is dependent on thecross-sectional area of the windings and the area of the sliding contact. The output voltage is thus a serials of steps as the contact moves from one wire to next.Electrical noise may be generated by variation in contact resistance, by mechanical wear due to contact friction, and by contact vibration transmitted from the sensing element. In addition, the motion being measured may experience significant mechanical loading by the inertia and friction of the moving parts of the potentiometer. The wear on the contacting surface limits the life of a potentiometer to a finite number of full strokes or rotations usually referred to in the manufacture’s specification as the ‘number of cycles of life expectancy’, a typical value being 20*1000000 cycles.The output voltage V0 of the unload potentiometer circuit is determined as follows. Let resistance R1= xi/xt *Rt where xi = input displacement, xt= maximum possible displacement, Rt total resistance of the potentiometer. Then output voltage V0= V*R1/(R1+( Rt-R1))=V*R1/Rt=V*xi/xt*Rt/Rt=V*xi/xt. This shows that there is a straight-line relationship between output voltage and input displacement for the unloaded potentiometer.It would seen that high sensitivity could be achieved simply by increasing the excitation voltage V. however, the maximum value of V is determined by the maximum power dissipation P of the fine wires of the potentiometer winding and is given by V=(PRt)1/2 .5.2 Resistance Strain GaugesResistance strain gauges are transducers which exhibit a change in electrical resistance in response to mechanical strain. They may be of the bonded or unbonded variety .a) bonded strain gaugesUsing an adhesive, these gauges are bonded, or cemented, directly on to the surface of the body or structure which is being examined.Examples of bonded gauges arei) fine wire gauges cemented to paper backingii) photo-etched grids of conducting foil on an epoxy-resin backingiii)a single semiconductor filament mounted on an epoxy-resin backing with copper or nickel leads.Resistance gauges can be made up as single elements to measuring strain in one direction only,or a combination of elements such as rosettes will permit simultaneous measurements in more than one direction.b) unbonded strain gaugesA typical unbonded-strain-gauge arrangement shows fine resistance wires stretched around supports in such a way that the deflection of the cantilever spring system changes the tension in the wires and thus alters the resistance of wire. Such an arrangement may be found in commercially available force, load, or pressure transducers.5.3 Resistance Temperature TransducersThe materials for these can be divided into two main groups:a) metals such as platinum, copper, tungsten, and nickel which exhibit and increase in resistance as the temperature rises; they have a positive temperature coefficient of resistance.b) semiconductors, such as thermistors which use oxides of manganese, cobalt, chromium, or nickel. These exhibit large non-linear resistance changes with temperature variation and normally have a negative temperature coefficient of resistance.a) metal resistance temperature transducersThese depend, for many practical purpose and within a narrow temperature range, upon the relationship R1=R0*[1+a*(b1-b2)] where a coefficient of resistance in ℃-1,and R0 resistance in ohms at the reference temperature b0=0℃ at the reference temperature range ℃.The international practical temperature scale is based on the platinum resistance thermometer, which covers the temperature range -259.35℃ to 630.5℃.b) thermistor resistance temperature transducersThermistors are temperature-sensitive resistors which exhibit large non-liner resistance changes with temperature variation. In general, they have a negative temperature coefficient. For small temperature increments the variation in resistance is reasonably linear; but, if large temperature changes are experienced, special linearizing techniques are used in the measuring circuits to produce a linear relationship of resistance against temperature.Thermistors are normally made in the form of semiconductor discs enclosed in glass vitreous enamel. Since they can be made as small as 1mm,quite rapid response times are possible.5.4 Photoconductive CellsThe photoconductive cell , uses a light-sensitive semiconductor material. The resistance between the metal electrodes decrease as the intensity of the light striking the semiconductor increases. Common semiconductor materials used for photo-conductive cells are cadmium sulphide, lead sulphide, and copper-doped germanium.The useful range of frequencies is determined by material used. Cadmium sulphide is mainly suitable for visible light, whereas lead sulphide has its peak response in the infra-red regionand is, therefore , most suitable for flame-failure detection and temperature measurement. 5.5 Photoemissive CellsWhen light strikes the cathode of the photoemissive cell are given sufficient energy to arrive the cathode. The positive anode attracts these electrons, producing a current which flows through resistor R and resulting in an output voltage V.Photoelectrically generated voltage V=Ip.RlWhere Ip=photoelectric current(A),and photoelectric current Ip=Kt.BWhere Kt=sensitivity (A/im),and B=illumination input (lumen)Although the output voltage does give a good indication of the magnitude of illumination, the cells are more often used for counting or control purpose, where the light striking the cathode can be interrupted.6、Capacitive TransducersThe capacitance can thus made to vary by changing either the relative permittivity, the effective area, or the distance separating the plates. The characteristic curves indicate that variations of area and relative permittivity give a linear relationship only over a small range of spacings. Thus the sensitivity is high for small values of d. Unlike the potentionmeter, the variable-distance capacitive transducer has an infinite resolution making it most suitable for measuring small increments of displacement or quantities which may be changed to produce a displacement.7、Inductive TransducersThe inductance can thus be made to vary by changing the reluctance of the inductive circuit. Measuring techniques used with capacitive and inductive transducers:a)A.C. excited bridges using differential capacitors inductors.b)A.C. potentiometer circuits for dynamic measurements.c) D.C. circuits to give a voltage proportional to velocity for a capacitor.d) Frequency-modulation methods, where the change of C or L varies the frequency of an oscillation circuit.Important features of capacitive and inductive transducers are as follows:i)resolution infiniteii) accuracy+- 0.1% of full scale is quotediii)displacement ranges 25*10-6 m to 10-3miv) rise time less than 50us possibleTypical measurands are displacement, pressure, vibration, sound, and liquid level.8、Linear Variable-differential Ttransformer9、Piezo-electric Transducers10、Electromagnetic Transducers11、Thermoelectric Transducers12、Photoelectric Cells13、Mechanical Transducers and Sensing Elements传感器的基础知识传感器是一种把被测量转换为光的、机械的或者更平常的电信号的装置。
传感器外文翻译

毕业设计(论文)外文文献翻译院系:光电与通信工程年级专业:12电子信息工程姓名:刘燊学号:1106012133附件:Advances in Sensor Technology Development指导老师评语:指导教师签名:年月日——摘自夏伟强,樊尚春传感器技术的的新发展仪器仪表学报传感器技术的新进展传感器技术是新技术革命和信息社会的重要技术基础,是一门多学科交叉的科学技术,被公认为现代信息技术的源头。
近些年,传感器技术发展很快,取得了许多新进展,尤其在气体传感器、生物传感器、视觉传感器等方面取得了很多进展。
美国麻省理工学院华人科学家张曙光领导的研究小组借助一种特殊溶液,成功地找到了大规模制造嗅觉感受器的办法;同样是麻省理工学院的研究人员利用气相色谱-质谱技术感受识别气体分子,研制出一种能对微量有毒气体做出强烈反应的微型传感器;俄罗斯科学家以从一种普通蘑菇中提取的混合物为原料,与压电石英晶振构成谐振式传感器,能够探测空气中含量极低的酚成分;日本科学家研制出能快速识别流感病毒纳米传感器,有望以纳米技术为快速识别流感病毒、乙型肝炎病毒、疯牛病病原体和残留农药等物质提供新手段;西班牙巴塞罗那自治大学研制出新型缩微DNA分析传感器,这种传感器能将分析 DNA链的时间缩短到几分钟或几小时,智能仪器与传感器技术、空间生物智能传感技术。
可以在亲子鉴定到检测遗传修饰食物的一系列化验中应用,此外还能确定新药的遗传毒性;美国国家标准与技术研究院研发出一种超灵敏微型核磁共振(NMR)传感器,该微型传感器与微流体通道并列置于一个硅芯片之上,这项技术将核磁共振的探测灵敏度提升到一个新的台阶,将在化学分析中具有广泛的应用前景。
我国传感器技术虽然与国外相比还有很大差距,但近两年也取得了一些进展和突破,诞生了一些新产品,有些在国家重大型号工程中获得应用。
如资源环境技术领域中的环境监测及环境风险评价技术、大气复合污染关键气态污染物的快速在线监测技术和大气细粒子和超细粒子的快速在线监测技术,海洋技术领域中的海洋水质污染综合参数在线监测技术和海洋金属污染物现场和在线监测技术等。
电容式传感器的外文文献翻译、中英文翻译、外文翻译
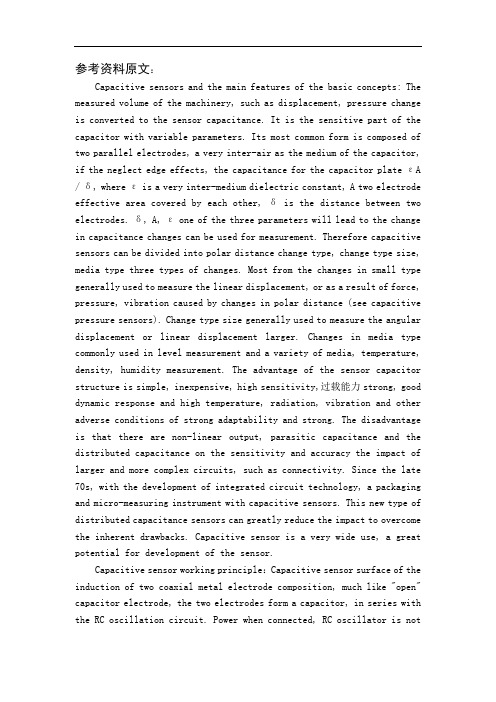
参考资料原文:Capacitive sensors and the main features of the basic concepts: The measured volume of the machinery, such as displacement, pressure change is converted to the sensor capacitance. It is the sensitive part of the capacitor with variable parameters. Its most common form is composed of two parallel electrodes, a very inter-air as the medium of the capacitor, if the neglect edge effects, the capacitance for the capacitor plate ε A / δ, where εis a very inter-medium dielectric constant, A two electrode effective area covered by each other, δ is the distance between two electrodes. δ, A, εone of the three parameters will lead to the change in capacitance changes can be used for measurement. Therefore capacitive sensors can be divided into polar distance change type, change type size, media type three types of changes.Most from the changes in small type generally used to measure the linear displacement, or as a result of force, pressure, vibration caused by changes in polar distance (see capacitive pressure sensors). Change type size generally used to measure the angular displacement or linear displacement larger. Changes in media type commonly used in level measurement and a variety of media, temperature, density, humidity measurement. The advantage of the sensor capacitor structure is simple, inexpensive, high sensitivity,过载能力strong, good dynamic response and high temperature, radiation, vibration and other adverse conditions of strong adaptability and strong. The disadvantage is that there are non-linear output, parasitic capacitance and the distributed capacitance on the sensitivity and accuracy the impact of larger and more complex circuits, such as connectivity. Since the late 70s, with the development of integrated circuit technology, a packaging and micro-measuring instrument with capacitive sensors.This new type of distributed capacitance sensors can greatly reduce the impact to overcome the inherent drawbacks. Capacitive sensor is a very wide use, a great potential for development of the sensor.Capacitive sensor working principle:Capacitive sensor surface of the induction of two coaxial metal electrode composition, much like "open" capacitor electrode, the two electrodes form a capacitor, in series with the RC oscillation circuit. Power when connected, RC oscillator is notoscillating, when a goal of moving around electrical capacitor, the capacitor capacity increased, the oscillator to start oscillation. Circuit after the passage of the deal, will be two kinds of vibration and vibration signals into switching signals, which played a detection purpose of the existence of any objects. The sensor can detect metal objects, but also to detect non-metallic objects, metal objects can move away from the largest, non-metallic objects on the decision to move away from the dielectric constant material, the greater the dielectric constant materials, the availability of action the greater distance.Application of capacitive sensors:Capacitive sensor can be used to measure linear displacement, angular displacement, vibration amplitude, especially suitable for measuring high-frequency vibration amplitude, precision rotary axis accuracy, acceleration and other mechanical parameters. Pole-changing type of application from a smaller displacement in the measurement range to several hundred microns in 0.01m, precision can reach 0.01m, a resolution of up to 0.001m. Change type size larger displacement can be measured, for the zero-range a few millimeters to a few hundred mm, 0.5 percent better than the linear resolution of 0.01 ~ 0.001m. Capacitive angular displacement sensor point of view and the dynamic range to a few degrees, a resolution of about 0.1 "up to the stability of the zero angle-second, widely used in precision angle measurement, such as for high-precision gyroscopes and accelerometers tilting . capacitive measurement sensor can measure the peak amplitude for the 0 ~ 50m, a frequency of 10 ~ 2kHz, sensitivity is higher than 0.01m, non-linear error of less than 0.05m.Capacitive sensor can also be used to measure pressure, differential pressure, level, surface, composition content (such as oil, the water content of food), non-metallic coating materials, such as film thickness, dielectric measurements of humidity, density, thickness, etc., in the automatic detection and control systems are also often used as a location signal generator. Capacitive differential pressure sensor measuring range up to 50MPa, an accuracy of ± 0.25% ~ ± 0.5%. Capacitive sensor for measuring range of the thickness of a few hundred microns, resolution of up to 0.01m. Capacitive Proximity Switches can not only detect metal, but also can detect plastic, wood,paper, and other dielectric liquids, but can not achieve the ultra-small, the movement distance of about 10 ~ 20mm. Electrostatic capacitive level switch is widely used in detection is stored in the tank, hopper, such as the location of containers in a variety of objects of a mature product. When the capacitive sensor measuring metal surface conditions, from the size, vibration amplitude is often used very variable from unilateral type, when the measured object is a capacitor electrode, and the other electrode in the sensor inside. This type of sensor is a non-contact measurement, dynamic range is relatively small, about a few millimeters is about the precision of more than 0.1m, a resolution of 0.01 ~ 0.001m.译文:电容式传感器的基本概念及主要特点:把被测的机械量,如位移、压力等转换为电容量变化的传感器。
测试信号分析与处理专业英语词汇
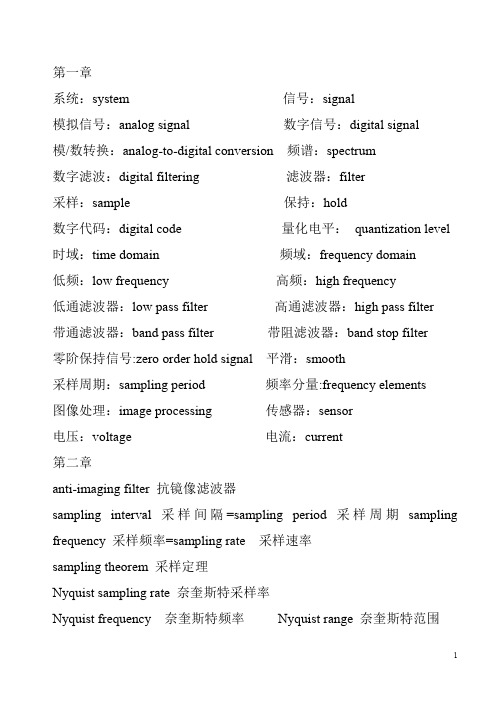
第一章系统:system 信号:signal模拟信号:analog signal 数字信号:digital signal模/数转换:analog-to-digital conversion 频谱:spectrum数字滤波:digital filtering 滤波器:filter采样:sample 保持:hold数字代码:digital code 量化电平:quantization level 时域:time domain 频域:frequency domain低频:low frequency 高频:high frequency低通滤波器:low pass filter 高通滤波器:high pass filter带通滤波器:band pass filter 带阻滤波器:band stop filter零阶保持信号:zero order hold signal 平滑:smooth采样周期:sampling period 频率分量:frequency elements图像处理:image processing 传感器:sensor电压:voltage 电流:current第二章anti-imaging filter 抗镜像滤波器sampling interval 采样间隔=sampling period 采样周期sampling frequency 采样频率=sampling rate 采样速率sampling theorem 采样定理Nyquist sampling rate 奈奎斯特采样率Nyquist frequency 奈奎斯特频率Nyquist range 奈奎斯特范围oversampling 过采样undersampling 欠采样quantization step 量化步长quantization noise量化噪声bit rate 比特率anti-aliasing filter 抗混叠滤波器第三章数字函数:digital function 合成函数:composite function 二维数字信号:two-dimensional digital signal语音信号:speech signal 量化方案:quantization scheme 脉冲函数:impulse function 单位脉冲函数:unit impulse function 阶跃函数:step function 幂函数:power function指数函数: exponential function 正弦函数:sine function余弦函数:cosine function 复平面:complex plain欧拉恒等式:Euler’s identity 模拟频率:analog frequency数字频率:digital frequency 采样间隔:sampling interval相移:phase shift 像素:pixel灰度级:gray scale第四章roll-off 滚降gain 增益pass band 通带stop band 阻带bandwidth 带宽linear system 线性系统superposition 叠加原理time-invariant 时不变causal system因果系统difference equation差分方程filter coefficient滤波器系数recursive filter 递归滤波器nonrecursive filter 非递归滤波器finite word length effect有限字长效应impulse response 脉冲响应infinite impulse response (IIR)无限脉冲响应finite impulse response (FIR)有限脉冲响应moving average filter 滑动平均滤波器step response 阶跃响应第六章z transform z变换region of convergence 收敛域inverse z transform 逆z变换transfer function 传输函数partial fraction expansion 部分分式展开cover-up method 覆盖法zero 零点pole 极点marginally stable 临界稳定unstable 不稳定第七章傅立叶变换:Fourier Transform 滤波器形状:filter shape频率响应:frequency response 频率特性:frequency characteristics 离散时间傅立叶变换:Discrete Time Fourier Transform幅度响应:magnitude response 相位响应:phase response传输函数:transfer function 相位差:phase difference采样频率:sampling frequency第八章white noise 白噪声magnitude spectrum 幅度频谱phase spectrum 相位频谱discrete Fourier series(DFS)离散傅里叶级数第九章有限脉冲响应滤波器:finite impulse response filter无限脉冲响应滤波器:infinite impulse response filter相位失真:phase distortion 理想低通滤波器:idle low pass filter 窗函数:window function 稳定性:stability通带波纹:pass band ripple 阻带波纹:stop band ripple通带边缘频率:pass band edge frequency过渡带宽度:transition width 矩形窗:Rectangular Window汉宁窗:Hanning Window 哈明窗:Hamming Window布莱克曼窗:Blackman Window 凯塞窗:Kaiser Window项数:number of terms 衰减:attenuation增益:gain 采样频率:sampling frequency第十章infinite impulse response filter(IIR)无限脉冲响应滤波器bilinear transformation 双线性变换prewarping equation 预扭曲方程Butterworth filter 巴特沃斯滤波器Chebyshev Type I filter 切比雪夫I 型滤波器Chebyshev Type II filter 切比雪夫II 型滤波器elliptic filter 椭圆滤波器Impulse invariance method 脉冲响应不变法。
检测与传感器技术(英文)

Sensors & Testing TechnologyCourse Code:80235000Course Name:Sensors & Testing TechnologyCredit:3.5 Semester to study:the 7th Teaching object:undergraduates in Mechanical Design and Manufacturing Automation major Pre-professional Courses:ElectrotechnicsCourse Director: Du qingfu,Associate professor,Master of ScienceCourse Description:The main task of this course is to enable students to master the basic principles of detection technology. It focuses on the basic knowledge of Sensors & Testing Technology. It also focuses on the working principle, the basic structure, the main performance measurement circuits and the application of sensors commonly used .The signal processing, interference suppression techniques and a number of new sensor detection system are also introduced in this course.Courses Assessment:The final results = usually results *30% + examination results *70%;Usually results are determined by attendance,homework,experiment performance and experimental report.Practice Teaching:Experiment is necessary to this course. Students need to preparate, carry out the experiments and write test report in accordance with the experiment guidance materials. And students also need to grasp the course knowledge and master the skill. Specified Teaching Materials:Qingfu, D. Sensors & Testing Technology( Ed.1). Shandong University Press,2008References:[1]Kejun X . Sensors & Testing Technology. Publishing House of Electronics Industry,2007[2]Xingpeng Z . Modern Testing Technology. Higher Education Press,2004[3]Zhiping X . Sensors & Testing Technology. Publishing House of Electronics Industry,2004。
数字通信中的多抽样率信号处理中英文翻译(部分)

数字通信中的多抽样率信号处理中英⽂翻译(部分)Multirate Signal Processing Concepts in Digital CommunicationsBojan VrceljIn Partial Fulfillment of the Requirementsfor the Degree ofDoctor of PhilosophyCalifornia Institute of TechnologyPasadena, California2004 (Submitted June 2, 2003)AbstractMultirate systems are building blocks commonly used in digital signal processing (DSP). Their function is to alter the rate of the discrete-time signals, which is achieved by adding or deleting a portion of the signal samples. Multirate systems play a central role in many areas of signal processing, such as filter bank theory and multiresolution theory. They are essential in various standard signal processing techniques such as signal analysis, denoising, compression and so forth. During the last decade, however, they have increasingly found applications in new and emerging areas of signal processing, as well as in several neighboring disciplines such as digital communications.The main contribution of this thesis is aimed towards better understanding of multirate systems and their use in modern communication systems. To this end, we first study a property of linear systems appearing in certain multirate structures. This property is called biorthogonal partnership and represents a terminology introduced recently to address a need for a descriptive term for such class of filters. In the thesis we especially focus on the extensions of this simple idea to the case of vector signals (MIMO biorthogonal partners) and to accommodate for nonintegral decimation ratios (fractional biorthogonal partners).Some of the main results developed here pertain to a better understanding of the biorthogonal partner relationship. These include the conditions for the existence of stable and of finite impulse response (FIR) biorthogonal partners. A major result that we establish states that under some generally mild conditions, MIMO and fractional biorthogonal partners exist. Moreover, when they exist, FIR solutions are not unique. We develop the parameterization of FIR solutions, which makes the search for the best partner in a given application analytically tractable. This proves very useful in the central application of biorthogonal partners, namely, channel equalization in digital communications with signal oversampling at the receiver. Sampling the received signal at a rate higher than that defined by the transmitter provides some flexibility in the design of the equalizer. A good channel equalizer in this context is one that helps neutralize the distortion on the signal introduced by the channel propagation but not at the expense of amplifying the channel noise. This presents the rationale behind the partner design problem which is formulated and solved. Theperformance of such equalizers is then compared to several other equalization methods by computer simulations. These findings point to the conclusion that the communication system performance can be improved at the expense of an increased implementational cost of the receiver.While the multirate DSP in the aforementioned communication systems serves to provide additional degrees of freedom in the design of the receiver, another important class of multirate structures is used at the transmitter side in order to introduce the redundancy in the data stream. This redundancy generally serves to facilitate the equalization process by forcing certain structure on the transmitted signal. If the channel is unknown, this procedure helps to identify it; if the channel is ill-conditioned, additional redundancy helpsVavoid severe noise amplification at the receiver, and so forth. In the second part of the thesis, we focus on this second group of multirate systems, derive some of their properties and introduce certain improvements of the communication systems in question.We first consider the transmission systems that introduce the redundancy in the form of a cyclic prefix. The examples of such systems include the discrete multitone (DMT) and the orthogonal frequency division multiplexing (OFDM) systems. The cyclic prefix insertion helps to effectively divide the channel in a certain number of nonoverlaping frequency bands. We study the problem of signal precoding in such systems that serves to adjust the signal properties in order to fully take advantage of the channel and noise properties across different bands. Our ultimate goal is to improve the overall system performance by minimizing the noise power at the receiver. The special case of our general solution corresponds to the white channel noise and the best precoder under these circumstances simply performs the optimal power allocation.Finally, we study a different class of communication systems with induced signal redundancy, namely, the multiuser systems based on code division multiple access (CDMA). We specifically focus on the special class of CDMA systems called `a mutually orthogonal usercode receiver' (AMOUR). These systems use the transmission redundancy to facilitate the user separation at the receiver regardless of the (different) communication channels. While the method also guarantees the existence of the zero-forcing equalizers irrespective of the channel zero locations, the performance of these equalizers can be further improved by exploiting the inherent flexibility in their design. Weshow how to find the best equalizer from the class of zero-forcing solutions and then increase the size of this class by employing alternative sampling strategies at the receiver. Our method retains the separability properties of AMOUR systems while improving their robustness in the noisy environment.Chapter 1 IntroductionThe theory of multirate digital signal processing (DSP) has traditionally been applied to the contexts of filter banks [61], [13], [50] and wavelets [31], [72]. These play a very important role in signal decomposition, analysis, modeling and reconstruction. Many areas of signal processing would be hard to envision without the use of digital filter banks. This is especially true for audio, video and image compression, digital audio processing, signal denoising, adaptive and statistical signal processing. However, multirate DSP has recently found increasing application in digital communications as well. Multirate building blocks are the crucial ingredient in many modern communication systems, for example, the discrete multitone (DMT), digital subscriber line (DSL) and the orthogonal frequency division multiplexing (OFDM) systems as well as general filter bank precoders, just to name a few. The interested reader is referred to numerous references on these subjects, such as [7]-[9], [17]-[18], [27], [30], [49], [64], [89], etc.This thesis presents a contribution to further understanding of multirate systems and their significance in digital communications. To that end, we introduce some new signal processing concepts and investigate their properties. We also consider some important problems in communications especially those that can be formulated using the multirate methodology. In this introductory chapter, we give a brief overview of the multirate systems and introduce some identities, notations and terminology that will prove useful in the rest of the thesis. Every attempt is made to make the present text as self-contained as possible and the introduction is meant to primarily serve this purpose. While some parts of the thesis, especially those that cover the theory of biorthogonal partners and their extensions provide a rather extensive treatment of the concepts, the material regarding the applications of the multirate theory in communication systems should be viewed as a contribution to a better understanding and by no means the exhaustive treatment of such systems. For a more comprehensive coverage the reader is referred to a range of extensive texts on the subject, for example, [71], [18], [19], [39], [38], [53], etc.1.1 Multirate systems 1.1.1 Basic building blocks The signals of interest in digital signal processing are discrete sequences of real or complex numbers denoted by x(n), y(n), etc. The sequence x(n) is often obtained by sampling a continuous-time signal x c(t). The majority of natural signals (like the audio signal reaching our ears or the optical signal reaching our eyes) are continuous-time. However, in order to facilitate their processing using DSP techniques, they need to be sampled and converted to digital signals. This conversion also includes signal quantization, i.e.,discretization in amplitude, however in practice it is safe to assume that the amplitude of x(n) can be any real or complexSignal processing analysis is often simplified by considering the frequency domain representation of signals and systems. Commonly used alternative representations of x(n) are its z-transform X (z) and the discrete-time Fourier transform X (O'). The z-transform is defined as X(z) = E _.x(n)z-"', and X (e j") is nothing but X(z) evaluated on the unit circle z = e3".Multirate DSP systems are usually composed of three basic building blocks, operating on a discrete-time signal x(n). Those are the linear time invariant (LTI) filter, the decimator and the expander. An LTI filter, like the one shown in Fig.1.1, is characterized by its impulse response h(n), or equivalently by its z-transform (also called the transfer function) H(z). Examples of the M-fold decimator and expander for M = 2 are shown in Fig.1.2. The rate of the signal at the output of an expander is M times higher than the rate at its input, while the converse is true for decimators. That is why the systems containing expanders and decimators are called `multirate' systems. Fig.1.2 demonstrates the behavior of the decimator andthe expander in both the time and the frequency domains.XE(z) = [X (z)]IM XD(z) = [X (z)]iM = X(z M)1 M-1 1 j2 k =M E X(z e n a)k=0for M-fold expander, and (1.1)for M-fold decimator. (1.2)The systems shown in Figs.1.1 and 1.2 operate on scalar signals and thus are called single input-single output (SISO) systems. The extensions to the case of vector signals are ratherstraightforward: the decimation and the expansion are performed on each element separately. The corresponding vector sequence decimators/expanders are denoted within square boxes in block diagrams. In Fig.1.3 this is demonstrated for vector expanders. The LTI systems operating on vector signals are called multiple input-multiple output (MIMO) systems and they are characterized by a (possibly rectangular) matrix transfer function H(z).1.1.2 Some multirate definitions and identitiesThe vector signals are sometimes obtained from the corresponding scalar signals by blocking. Conversely, the scalar signals can be recovered from the vector signals by unblocking. The blocking/unblocking operations can be defined using the delay or the advance chains [61], thus leading to two similar definitions. One way of defining these operations is shown in Fig.1.4, while the other is obtained trivially by switching the delay and the advance operators. Instead of drawing the complete delay/advance chain structure, we often use the simplified block notation as in Fig.1.4. It is usually clear from the context which of the two definitions数字通信中的多抽样率信号处理Bojan Vrcelj博⼠学位论⽂加州技术学会Pasadena, 加州2004 (委托于2003.6.2)摘要多抽样率系统普遍是被运⽤在处理数字信号⽅⾯。
传感器 外文翻译 外文文献 英文文献 分布是温度传感器
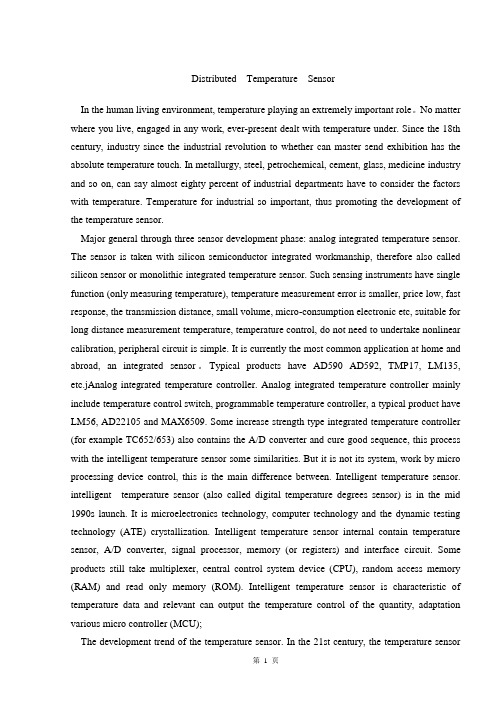
Distributed Temperature SensorIn the human living environment, temperature playing an extremely important role。
No matter where you live, engaged in any work, ever-present dealt with temperature under. Since the 18th century, industry since the industrial revolution to whether can master send exhibition has the absolute temperature touch. In metallurgy, steel, petrochemical, cement, glass, medicine industry and so on, can say almost eighty percent of industrial departments have to consider the factors with temperature. Temperature for industrial so important, thus promoting the development of the temperature sensor.Major general through three sensor development phase: analog integrated temperature sensor. The sensor is taken with silicon semiconductor integrated workmanship, therefore also called silicon sensor or monolithic integrated temperature sensor. Such sensing instruments have single function (only measuring temperature), temperature measurement error is smaller, price low, fast response, the transmission distance, small volume, micro-consumption electronic etc, suitable for long distance measurement temperature, temperature control, do not need to undertake nonlinear calibration, peripheral circuit is simple. It is currently the most common application at home and abroad, an integrated sensor。
传感器与检测技术英文书籍英语

传感器与检测技术英文书籍英语Sensors and Detection Technologies.Sensors and detection technologies are essential components of modern instrumentation and control systems. They provide the means to measure and monitor physical, chemical, and biological parameters, and transmit this information to other devices for processing and analysis.There is a wide variety of sensors and detection technologies available, each with its own unique set of capabilities and limitations. The choice of sensor for a particular application depends on factors such as the parameter to be measured, the desired accuracy and precision, the operating environment, and the cost.Some of the most common types of sensors include:Temperature sensors measure the temperature of a substance. They can be based on a variety of principles,including thermocouples, resistance temperature detectors (RTDs), and thermistors.Pressure sensors measure the pressure of a gas or liquid. They can be based on a variety of principles, including strain gauges, diaphragms, and piezoresistive elements.Flow sensors measure the flow rate of a gas or liquid. They can be based on a variety of principles, including differential pressure, thermal dispersion, and ultrasonic waves.Level sensors measure the level of a liquid or solidin a tank or other container. They can be based on avariety of principles, including float switches, ultrasonic waves, and capacitance probes.Gas sensors measure the concentration of a gas in a sample. They can be based on a variety of principles, including electrochemical cells, semiconductor sensors, and optical sensors.Chemical sensors measure the concentration of a chemical species in a sample. They can be based on avariety of principles, including ion-selective electrodes, potentiometric sensors, and amperometric sensors.Biological sensors measure the presence or concentration of a biological molecule in a sample. Theycan be based on a variety of principles, including immunoassays, DNA hybridization, and protein binding assays.Detection technologies are used to convert the outputof a sensor into a digital signal that can be processed and analyzed by a computer or other device. Some of the most common types of detection technologies include:Analog-to-digital converters (ADCs) convert an analog signal into a digital signal.Digital-to-analog converters (DACs) convert a digital signal into an analog signal.Counters count the number of pulses or events that occur over a period of time.Timers measure the duration of a period of time.Data acquisition systems collect and store data from sensors and other devices.Sensors and detection technologies are used in a wide variety of applications, including:Industrial automation.Medical diagnostics.Environmental monitoring.Military and defense.Scientific research.The development of new sensors and detectiontechnologies is an active area of research and development. New sensors are being developed to measure a wider range of parameters with greater accuracy and precision. New detection technologies are being developed to improve the signal-to-noise ratio and reduce the cost of data acquisition systems.The continued development of sensors and detection technologies will enable new and innovative applications in a wide variety of fields.。
《信号与系统》专业术语中英文对照表
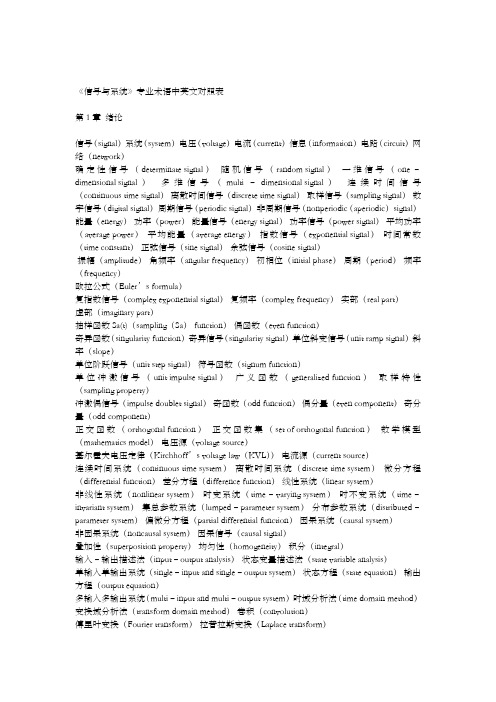
《信号与系统》专业术语中英文对照表第 1 章绪论信号(signal)系统(system)电压(voltage)电流(current)信息(information)电路(circuit)网络(network)确定性信号(determinate signal)随机信号(random signal)一维信号(one–dimensional signal)多维信号(multi–dimensional signal)连续时间信号(continuous time signal)离散时间信号(discrete time signal)取样信号(sampling signal)数字信号(digital signal)周期信号(periodic signal)非周期信号(nonperiodic(aperiodic) signal)能量(energy)功率(power)能量信号(energy signal)功率信号(power signal)平均功率(average power)平均能量(average energy)指数信号(exponential signal)时间常数(time constant)正弦信号(sine signal)余弦信号(cosine signal)振幅(amplitude)角频率(angular frequency)初相位(initial phase)周期(period)频率(frequency)欧拉公式(Euler’s formula)复指数信号(complex exponential signal)复频率(complex frequency)实部(real part)虚部(imaginary part)抽样函数 Sa(t)(sampling(Sa) function)偶函数(even function)奇异函数(singularity function)奇异信号(singularity signal)单位斜变信号(unit ramp signal)斜率(slope)单位阶跃信号(unit step signal)符号函数(signum function)单位冲激信号(unit impulse signal)广义函数(generalized function)取样特性(sampling property)冲激偶信号(impulse doublet signal)奇函数(odd function)偶分量(even component)奇分量(odd component)正交函数(orthogonal function)正交函数集(set of orthogonal function)数学模型(mathematics model)电压源(voltage source)基尔霍夫电压定律(Kirchhoff’s voltage law(KVL))电流源(current source)连续时间系统(continuous time system)离散时间系统(discrete time system)微分方程(differential function)差分方程(difference function)线性系统(linear system)非线性系统(nonlinear system)时变系统(time–varying system)时不变系统(time–invariant system)集总参数系统(lumped–parameter system)分布参数系统(distributed–parameter system)偏微分方程(partial differential function)因果系统(causal system)非因果系统(noncausal system)因果信号(causal signal)叠加性(superposition property)均匀性(homogeneity)积分(integral)输入–输出描述法(input–output analysis)状态变量描述法(state variable analysis)单输入单输出系统(single–input and single–output system)状态方程(state equation)输出方程(output equation)多输入多输出系统(multi–input and multi–output system)时域分析法(time domain method)变换域分析法(transform domain method)卷积(convolution)傅里叶变换(Fourier transform)拉普拉斯变换(Laplace transform)第 2 章连续时间系统的时域分析齐次解(homogeneous solution)特解(particular solution)特征方程(characteristic function)特征根(characteristic root)固有(自由)解(natural solution)强迫解(forced solution)起始条件(original condition)初始条件(initial condition)自由响应(natural response)强迫响应(forced response)零输入响应(zero-input response)零状态响应(zero-state response)冲激响应(impulse response)阶跃响应(step response)卷积积分(convolution integral)交换律(exchange law)分配律(distribute law)结合律(combine law)第3 章傅里叶变换频谱(frequency spectrum)频域(frequency domain)三角形式的傅里叶级数(trigonomitric Fourier series)指数形式的傅里叶级数(exponential Fourier series)傅里叶系数(Fourier coefficient)直流分量(direct composition)基波分量(fundamental composition) n 次谐波分量(nth harmonic component)复振幅(complex amplitude)频谱图(spectrum plot(diagram))幅度谱(amplitude spectrum)相位谱(phase spectrum)包络(envelop)离散性(discrete property)谐波性(harmonic property)收敛性(convergence property)奇谐函数(odd harmonic function)吉伯斯现象(Gibbs phenomenon)周期矩形脉冲信号(periodic rectangular pulse signal)周期锯齿脉冲信号(periodic sawtooth pulse signal)周期三角脉冲信号(periodic triangular pulse signal)周期半波余弦信号(periodic half–cosine signal)周期全波余弦信号(periodic full–cosine signal)傅里叶逆变换(inverse Fourier transform)频谱密度函数(spectrum density function)单边指数信号(single–sided exponential signal)双边指数信号(two–sided exponential signal)对称矩形脉冲信号(symmetry rectangular pulse signal)线性(linearity)对称性(symmetry)对偶性(duality)位移特性(shifting)时移特性(time–shifting)频移特性(frequency–shifting)调制定理(modulation theorem)调制(modulation)解调(demodulation)变频(frequency conversion)尺度变换特性(scaling)微分与积分特性(differentiation and integration)时域微分特性(differentiation in the time domain)时域积分特性(integration in the time domain)频域微分特性(differentiation in the frequency domain)频域积分特性(integration in the frequency domain)卷积定理(convolution theorem)时域卷积定理(convolution theorem in the time domain)频域卷积定理(convolution theorem in the frequency domain)取样信号(sampling signal)矩形脉冲取样(rectangular pulse sampling)自然取样(nature sampling)冲激取样(impulse sampling)理想取样(ideal sampling)取样定理(sampling theorem)调制信号(modulation signal)载波信号(carrier signal)已调制信号(modulated signal)模拟调制(analog modulation)数字调制(digital modulation)连续波调制(continuous wave modulation)脉冲调制(pulse modulation)幅度调制(amplitude modulation)频率调制(frequency modulation)相位调制(phase modulation)角度调制(angle modulation)频分多路复用(frequency–division multiplex(FDM))时分多路复用(time–division multiplex (TDM))相干(同步)解调(synchronous detection)本地载波(local carrier)系统函数(system function)网络函数(network function)频响特性(frequency response)幅频特性(amplitude frequency response)相频特性(phase frequency response)无失真传输(distortionless transmission)理想低通滤波器(ideal low–pass filter)截止频率(cutoff frequency)正弦积分(sine integral)上升时间(rise time)窗函数(window function)理想带通滤波器(ideal band–pass filter)第 4 章拉普拉斯变换代数方程(algebraic equation)双边拉普拉斯变换(two-sided Laplace transform)双边拉普拉斯逆变换(inverse two-sided Laplace transform)单边拉普拉斯变换(single-sided Laplace transform)拉普拉斯逆变换(inverse Laplace transform)收敛域(region of convergence(ROC))延时特性(time delay)s 域平移特性(shifting in the s-domain)s 域微分特性(differentiation in the s-domain) s 域积分特性(integration in the s-domain)初值定理(initial-value theorem)终值定理(expiration-value)复频域卷积定理(convolution theorem in the complex frequency domain)部分分式展开法(partial fraction expansion)留数法(residue method)第 5 章策动点函数(driving function)转移函数(transfer function)极点(pole)零点(zero)零极点图(zero-pole plot)暂态响应(transient response)稳态响应(stable response)稳定系统(stable system)一阶系统(first order system)高通滤波网络(high-low filter)低通滤波网络(low-pass filter)二阶系统(second system)最小相移系统(minimum-phase system)维纳滤波器(Winner filter)卡尔曼滤波器(Kalman filter)低通(low-pass)高通(high-pass)带通(band-pass)带阻(band-stop)有源(active)无源(passive)模拟(analog)数字(digital)通带(pass-band)阻带(stop-band)佩利-维纳准则(Paley-Winner criterion)最佳逼近(optimum approximation)过渡带(transition-band)通带公差带(tolerance band)巴特沃兹滤波器(Butterworth filter)切比雪夫滤波器(Chebyshew filter)方框图(block diagram)信号流图(signal flow graph)节点(node)支路(branch)输入节点(source node)输出节点(sink node)混合节点(mix node)通路(path)开通路(open path)闭通路(close path)环路(loop)自环路(self-loop)环路增益(loop gain)不接触环路(disconnect loop)前向通路(forward path)前向通路增益(forward path gain)梅森公式(Mason formula)劳斯准则(Routh criterion)第 6 章数字系统(digital system)数字信号处理(digital signal processing)差分方程(difference equation)单位样值响应(unit sample response)卷积和(convolution sum)Z 变换(Z transform)序列(sequence)样值(sample)单位样值信号(unit sample signal)单位阶跃序列(unit step sequence)矩形序列 (rectangular sequence)单边实指数序列(single sided real exponential sequence)单边正弦序列(single sided exponential sequence)斜边序列(ramp sequence)复指数序列(complex exponential sequence)线性时不变离散系统(linear time-invariant discrete-time system)常系数线性差分方程(linear constant-coefficient difference equation)后向差分方程(backward difference equation)前向差分方程(forward difference equation)海诺塔(Tower of Hanoi)菲波纳西(Fibonacci)冲激函数串(impulse train)第 7 章数字滤波器(digital filter)单边 Z 变换(single-sided Z transform)双边 Z 变换(two-sided (bilateral) Z transform) 幂级数(power series)收敛(convergence)有界序列(limitary-amplitude sequence)正项级数(positive series)有限长序列(limitary-duration sequence)右边序列(right-sided sequence)左边序列(left-sided sequence)双边序列(two-sided sequence) Z 逆变换(inverse Z transform)围线积分法(contour integral method)幂级数展开法(power series expansion) z 域微分(differentiation in the z-domain)序列指数加权(multiplication by an exponential sequence) z 域卷积定理(z-domain convolution theorem)帕斯瓦尔定理(Parseval theorem)传输函数(transfer function)序列的傅里叶变换(discrete-time Fourier transform:DTFT)序列的傅里叶逆变换(inverse discrete-time Fourier transform:IDTFT)幅度响应(magnitude response)相位响应(phase response)量化(quantization)编码(coding)模数变换(A/D 变换:analog-to-digital conversion)数模变换(D/A 变换:digital-to- analog conversion)第 8 章端口分析法(port analysis)状态变量(state variable)无记忆系统(memoryless system)有记忆系统(memory system)矢量矩阵(vector-matrix )常量矩阵(constant matrix )输入矢量(input vector)输出矢量(output vector)直接法(direct method)间接法(indirect method)状态转移矩阵(state transition matrix)系统函数矩阵(system function matrix)冲激响应矩阵(impulse response matrix)朱里准则(July criterion)。
传感器英语
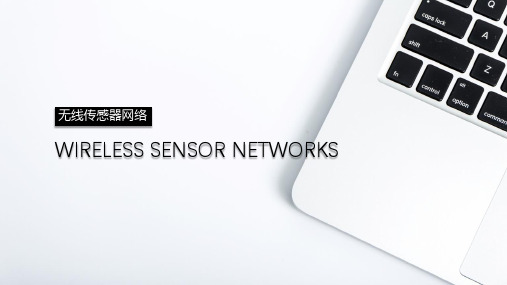
当这些设备部署在一个广泛的地理区域时,它们可以收集关于环 境的信息,并通过形成一个分布式通信网络,既无线传感器网络 (WSM)有效地协作处理这些信息,如图1.1所示。
A WSN is a special case of an ad-hoc wireless network, and assumes a multi-hop communication framework with no common infrastructure, where the sensors spontaneously cooperate to deliver information by forwarding packets from a source to a destination.
无线传感器网络
WIRELESS SENSOR NETWORKS
vocabulary
sensor
[ˈsensə(r)]
deploy
[dɪˈplɔɪ]
collaborate
[kəˈlæ bəreɪt]
illustrate
[ˈɪləstreɪt]
status
[ˈsteɪtəs]
传感器技术外文文献及中文翻译
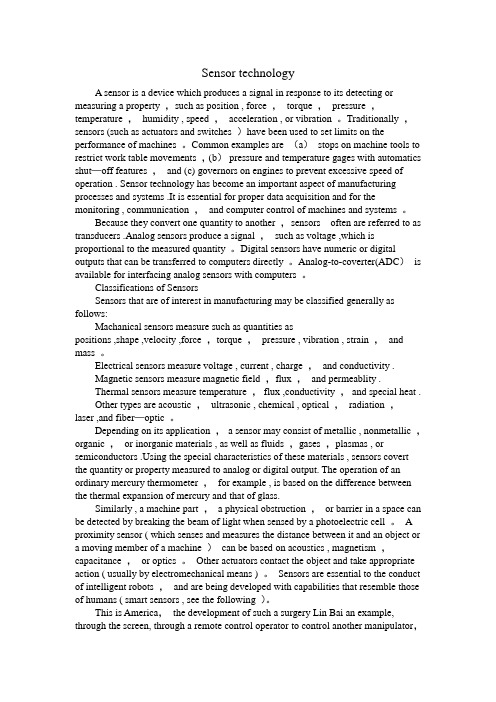
Sensor technologyA sensor is a device which produces a signal in response to its detecting or measuring a property ,such as position , force ,torque ,pressure ,temperature ,humidity , speed ,acceleration , or vibration 。
Traditionally ,sensors (such as actuators and switches )have been used to set limits on the performance of machines 。
Common examples are (a)stops on machine tools to restrict work table movements ,(b)pressure and temperature gages with automatics shut—off features ,and (c) governors on engines to prevent excessive speed of operation . Sensor technology has become an important aspect of manufacturing processes and systems .It is essential for proper data acquisition and for the monitoring , communication ,and computer control of machines and systems 。
Because they convert one quantity to another ,sensors often are referred to as transducers .Analog sensors produce a signal ,such as voltage ,which is proportional to the measured quantity 。
传感器技术外文文献及中文翻译
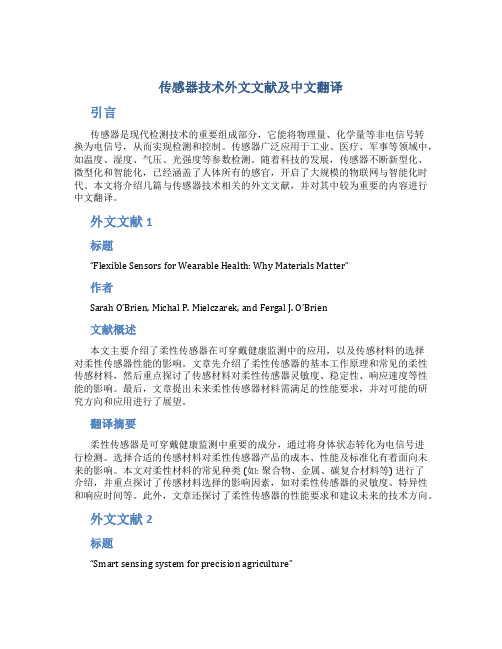
传感器技术外文文献及中文翻译引言传感器是现代检测技术的重要组成部分,它能将物理量、化学量等非电信号转换为电信号,从而实现检测和控制。
传感器广泛应用于工业、医疗、军事等领域中,如温度、湿度、气压、光强度等参数检测。
随着科技的发展,传感器不断新型化、微型化和智能化,已经涵盖了人体所有的感官,开启了大规模的物联网与智能化时代。
本文将介绍几篇与传感器技术相关的外文文献,并对其中较为重要的内容进行中文翻译。
外文文献1标题“Flexible Sensors for Wearable Health: Why Materials Matter”作者Sarah O’Brien, Michal P. Mielczarek, and Fergal J. O’Brien文献概述本文主要介绍了柔性传感器在可穿戴健康监测中的应用,以及传感材料的选择对柔性传感器性能的影响。
文章先介绍了柔性传感器的基本工作原理和常见的柔性传感材料,然后重点探讨了传感材料对柔性传感器灵敏度、稳定性、响应速度等性能的影响。
最后,文章提出未来柔性传感器材料需满足的性能要求,并对可能的研究方向和应用进行了展望。
翻译摘要柔性传感器是可穿戴健康监测中重要的成分,通过将身体状态转化为电信号进行检测。
选择合适的传感材料对柔性传感器产品的成本、性能及标准化有着面向未来的影响。
本文对柔性材料的常见种类 (如: 聚合物、金属、碳复合材料等) 进行了介绍,并重点探讨了传感材料选择的影响因素,如对柔性传感器的灵敏度、特异性和响应时间等。
此外,文章还探讨了柔性传感器的性能要求和建议未来的技术方向。
外文文献2标题“Smart sensing system for precision agriculture”作者Olivier Strauss, Lucas van der Meer, and Benoit Figliuzzi文献概述本文主要介绍智能传感系统在精准农业中的应用。
多传感器信号检测与分析算法研究
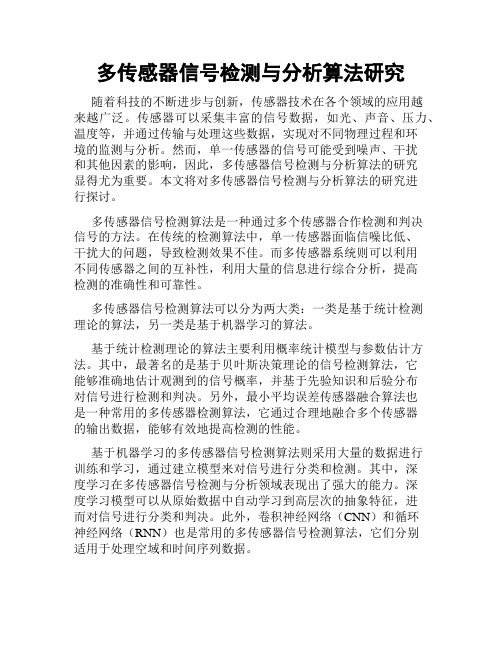
多传感器信号检测与分析算法研究随着科技的不断进步与创新,传感器技术在各个领域的应用越来越广泛。
传感器可以采集丰富的信号数据,如光、声音、压力、温度等,并通过传输与处理这些数据,实现对不同物理过程和环境的监测与分析。
然而,单一传感器的信号可能受到噪声、干扰和其他因素的影响,因此,多传感器信号检测与分析算法的研究显得尤为重要。
本文将对多传感器信号检测与分析算法的研究进行探讨。
多传感器信号检测算法是一种通过多个传感器合作检测和判决信号的方法。
在传统的检测算法中,单一传感器面临信噪比低、干扰大的问题,导致检测效果不佳。
而多传感器系统则可以利用不同传感器之间的互补性,利用大量的信息进行综合分析,提高检测的准确性和可靠性。
多传感器信号检测算法可以分为两大类:一类是基于统计检测理论的算法,另一类是基于机器学习的算法。
基于统计检测理论的算法主要利用概率统计模型与参数估计方法。
其中,最著名的是基于贝叶斯决策理论的信号检测算法,它能够准确地估计观测到的信号概率,并基于先验知识和后验分布对信号进行检测和判决。
另外,最小平均误差传感器融合算法也是一种常用的多传感器检测算法,它通过合理地融合多个传感器的输出数据,能够有效地提高检测的性能。
基于机器学习的多传感器信号检测算法则采用大量的数据进行训练和学习,通过建立模型来对信号进行分类和检测。
其中,深度学习在多传感器信号检测与分析领域表现出了强大的能力。
深度学习模型可以从原始数据中自动学习到高层次的抽象特征,进而对信号进行分类和判决。
此外,卷积神经网络(CNN)和循环神经网络(RNN)也是常用的多传感器信号检测算法,它们分别适用于处理空域和时间序列数据。
除了传感器信号检测,多传感器信号分析算法也是非常重要的一部分。
传感器数据的分析可以帮助我们深入理解各类物理过程和环境,并从中挖掘有用的信息。
多传感器信号分析算法可以通过对多个传感器数据的特征提取、相互关联性分析、时频分析等方法,实现对信号的理解和描述。
传感器专业英语词汇
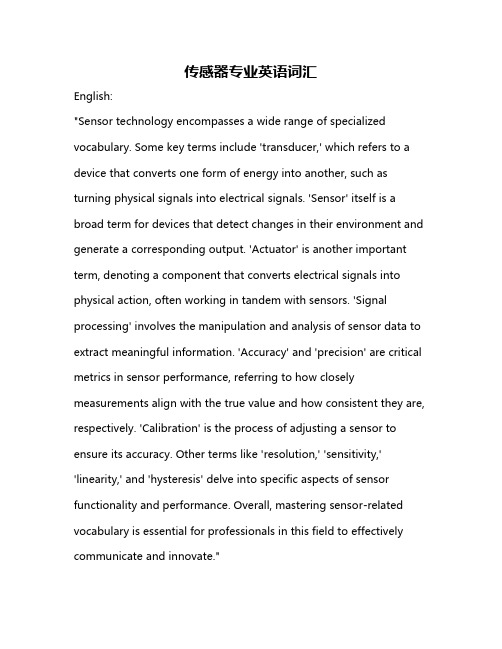
传感器专业英语词汇English:"Sensor technology encompasses a wide range of specialized vocabulary. Some key terms include 'transducer,' which refers to a device that converts one form of energy into another, such as turning physical signals into electrical signals. 'Sensor' itself is a broad term for devices that detect changes in their environment and generate a corresponding output. 'Actuator' is another important term, denoting a component that converts electrical signals into physical action, often working in tandem with sensors. 'Signal processing' involves the manipulation and analysis of sensor data to extract meaningful information. 'Accuracy' and 'precision' are critical metrics in sensor performance, referring to how closely measurements align with the true value and how consistent they are, respectively. 'Calibration' is the process of adjusting a sensor to ensure its accuracy. Other terms like 'resolution,' 'sensitivity,''linearity,' and 'hysteresis' delve into specific aspects of sensor functionality and performance. Overall, mastering sensor-related vocabulary is essential for professionals in this field to effectively communicate and innovate."中文翻译:"传感器技术涵盖了广泛的专业词汇。
- 1、下载文档前请自行甄别文档内容的完整性,平台不提供额外的编辑、内容补充、找答案等附加服务。
- 2、"仅部分预览"的文档,不可在线预览部分如存在完整性等问题,可反馈申请退款(可完整预览的文档不适用该条件!)。
- 3、如文档侵犯您的权益,请联系客服反馈,我们会尽快为您处理(人工客服工作时间:9:00-18:30)。
外文翻译毕业设计题目:多传感器检测系统信号处理研究原文1:911plus CTD SYSTEM OPERATINGAND REPAIR MANUAL 5-1译文1:911plus CTD系统的操作和维修手册5-1原文2:911plus CTD SYSTEM OPERATINGAND REPAIR MANUAL 5-2译文2:911plus CTD系统的操作和维修手册5-2 1-3TROUBLE DIAGNOSIS AND REPAIR (UNDERWATER UNITPROBLEMS)(原文1) LIFE-THREATENING HIGH VOLTAGES ARE PRESENT IN BOTH DECK UNIT AND UNDERW ATER UNIT WHEN POWER IS ON. THESE HAZARDOUS VOLTAGES PERSIST FOR UP TO ONE MINUTE AFTER REMOV AL OF POWER..THE BEST W AY TO PROTECT AGAINST ELECTRICAL SHOCK IS TO DISCONNECT THE AC POWER CORDS FROM THE REAR PANEL OF THE DECK UNIT, THEN WAIT A FULL MINUTE BEFORE ATTEMPTING SERVICE.ALWAYS DISCONNECT THE AC POWER CORD BEFORE CHECKING FUSES!!!For protection of the circuitry, we also recommend that AC power be removed and a 1 minute period for supply capacitor discharge be allowed before opening housings, changing connections, removing or inserting circuit cards, or otherwise working on the equipment.If the data telemetry is OK (deck unit data light is on and error light off) but one or more channels is faulty, see Section 5-2 (Sensor Problems) before proceeding with work on the underwater unit; the problem may be a defective sensor.Read Section 4-1 which describes the operation of the underwater unit circuitry. Refer also to the section titled 'schematics' which contains the underwater unit schematic diagrams.All voltages are measured relative to pin 2 of any plug-in card; this is main signal/power ground for the underwater unit.5-1.1 UNDERW ATER UNIT COMPLETELY INOPERATIVEMake sure that the deck unit is supplying the proper sea cable voltage (250 volts). If there is no sea-cable voltage, the seacable rear panel fuse may be blown.With SEARAM units, see that SEARAM is supplying the proper voltage to the CTD (12 - 15 volts).Look for the presence of the telemetry waveform across the sea cable. USE EXTREME CAUTION IN PERFORMING THIS OBSERV ATION. THE SEA CABLE VOLTAGE IS POTENTIALL Y LIFE-THREATENING!ALLOW 1 MINUTE AFTER POWER-DOWN FOR POWER SUPPL Y DISCHARGE BEFORE MAKING CONNECTIONS TO THE SEA CABLE OUTPUT CONNECTOR. If the telemetrywaveform is present, the trouble is probably in the deck unit.If the telemetry waveform is not present at the deck unit, measure the sea cable current which will be in the range of 50 to 220 milliamperes depending on the underwater unit configuration.If the sea cable voltage is present, but no current is being drawn by the underwater unit, there is either a break in the sea cable, or an open circuit in the cable interface circuitry inside the underwater unit.Assuming that there is no break in the sea cable, turn off the deck unit power, refer to Section 6-1 and open the Underwater Unit housing, but leave the electronics chassis connected to the top end cap.Restore deck unit power and measure the internal supply voltages (relative to pin 2): +5, +15 and -15 at pins 1, 5, and 3 respectively of the Analog interface boards.If there is no voltage at any point on the backplane, the sea cable interface circuitry is either defective or is not receiving power from the sea cable. Check that the Sea-cable Interface is receiving the correct input voltage (250 volts).If no voltage is present at the inputs to the DC/DC converters, the series pass transistor Q1 on the sea-cable interface board may be open.If the +5, +8, +15, or -15 volt levels are absent or in error by more than about 0.5 volts, remove all the underwater unit plug in printed circuit boards. Check the power supply levels again - if these are now ok, the problem is probably in one of the plug-in boards. Start plugging boards in, beginning with the Transmitter (top) board. (Turn off power and wait one minute for supply discharge before plugging in each board). If a board is found which appears to cause the drop in supply voltage, refer to the circuit description in Section 4-1 and the board's schematic diagram.Check the board for dead shorts at the power input pins -an IC or one of the power supply bypass capacitors may be shorted. Also, carefully examine the board for any metallic material (solder, wire clippings, shavings) which may have inadvertently shorted the printed circuit traces or component pins.With the board in the underwater unit chassis, look at the board outputs and inputs for signal irregularities. The logic levels are 5 volt CMOS and should swing the full 0 to +5 volts.If the power supply levels are not ok with the plug-in boards removed, check the backplane wiring for broken connections or wires, and for any shorted connections. Check that the inputvoltage to the DC/DC converters is correct. If so, one of the converters may be defective, or one of the by-pass capacitors across the converter outputs may be shorted.5-1.2 POWER SUPPLIES OK BUT NO DATACheck Logic Board pin 3 for the NRZ logic level. If this signal is present, check phase-reversing output at Transmitter Board pin 8.If the phase-encoded signal is present at the Transmitter Board output, the problem is in the transformer coupling to the sea-cable, or in the sea-cable wiring itself.If the phase-encoded signal is missing (or wrong in frequency or waveform), check for proper output signals from the Logic 1 Board. The following should be observed:Pin 5 FR 27,648 kHz (square wave)Pin 4 FR/4 6,912 kHz (square wave)Pin H 69120 Hz (square wave)Pin 6 8640 (square wave)If the signals described above are ok on the Logic Board alone, reinstall the remaining circuit boards one-by-one, checking the suspect signal after each card is installed until the faulty card is located.5-1.3 ONE OR MORE NON-FUNCTIONING CHANNELSIf a frequency channel is giving improper readings, check that the sensor signal is present on AP counter board (pins 7 and H on the backplane, found on the right edge of the small board that the coax cabling is mounted to). These are 2 volt p-p sine waves in the frequency range of 2800 - 12000 Hz for temperature and conductivity and a 4 volt square wave in the range 35000 to 40000 Hz for the pressure sensor.If one of the sensor outputs is faulty, remove that channel's AP Counter Board - if this restores the signal, the AP Board has a defective input. Try one of the other AP Counters (the five boards are identical) in the offending channel position.If the AP Counter inputs are ok, try swapping AP Counter Boards to locate the faulty one. If the problem stays with the same channel, check that the FR, SE, SC, and RESET, inputs are active.If the problem is a faulty A/D channel, check that the inputs to the A/D Board multiplexer (pins D, F, H, J, L, 10, 8, 7) are as assumed. Check for +15 and -15 volts at the A/D Board. Lookfor the SE scan clock at pin 9; this starts the (rapid ~ 50 μs/channel) acquisition of A/D channel data. Check that the SC shift clock is present at pin 6, and that serial data is present at pin 4.5-1.4 PUMP NOT WORKINGCTDs shipped after 1 January 1990 have a "hard-wired" pump delay to facilitate pump priming. With this feature, the pump turn-on is enabled only after two conditions are met. First, the conductivity cell must be filled with seawater which causes the conductivity frequency to rise above the 0 conductivity frequency. Second, when the CTD senses the proper increase in frequency, it starts a 60 second delay timer. This delay allows sufficient time for the air in the tubing to escape through the air bleed-hole. Be sure to hold the pump just under the surface with the top of the tubing underwater for at least a full minute before beginning the profile.Note: The control logic for this feature only functions on the "Primary" conductivity channel. If the CTD is equipped with redundant T & C sensors and pumps, and you wish to deploy it with the "Primary" conductivity sensor removed, be sure to swap the "Secondary" T & C sensor pair to the "Primary" T & C channel bulkhead connectors.Note: with old water sampler systems that interrupt CTD power when tripping a bottle, wait 60 seconds after bottle firing for the pump to restart (it is not necessary to wait when using the Sea-Bird subcarrier modem / rosette interface system).Make sure that the pump cable is not cut or otherwise damaged and that the rubber molded plug is fully mated to the pump housing connector. Unplug the pump from its external cable. Apply +12 volts to the pump housing's small connector pin, return to the large pin. Look for a current drain of about 300 milliamperes and listen for the pump running. If the pump passes this test, the problem is in the main housing or in the cabling. Disassemble the underwater unit and perform the following tests.Unplug the conductivity sensor. Connect a audio signal generator (approximately 2 volts p-p output) at pin 7 (return to pin H) of the AP Counter Board for primary conductivity (C1). Set the generator frequency to about 2500 Hz. Observe the squared signal at U2A output. Measure the voltage at pin L which should be zero. Increase the generator frequency to about 4000 Hz. The voltage at pin L should now be 5 volts. If not, the one-shot U3B or the buffer U2B is defective.If the voltage at C1 AP Counter Board pin L responds properly, make sure that it also appears at the Transmitter Board pin K. 60 seconds after Transmitter Board pin K goes high, pin L shouldalso go high (to nearly 15 volts). If this is the case, the problem is in the wiring to the pump external connector.作者:SEA-BIRD电子股份有限公司国籍:美国出处:SEA-BIRD电子股份有限公司SENSOR PROBLEMS(原文2):Apparent sensor problems may be the result of trouble with the cables or the acquisition circuitry in the underwater unit, or the trouble may be nothing more than an incorrect entry of the sensor calibration coefficients. The conductivity and temperature sensors have identical power and output characteristics, and may be freely interchanged to help localize the fault.Although repair of these sensors is certainly possible, it is not likely that repairs can be made without affecting the sensor's calibration. The Sea-Bird sensors are small and easily replaced in the field; as they are supplied with calibration coefficients, a spare sensor can get a failed unit into fully operating and calibrated condition with a minimum of trouble. Sea-Bird is also always ready to send replacement sensors by air courier immediately upon notification of a failure.5-2.1 CONDUCTIVITY AND TEMPERATURE SENSORSIf a sensor is generating conductivity, temperature, or pressure-dependent frequency but the indicated value is significantly erroneous, check that the coefficients used in the processing software are as stated on the calibration certificates supplied with the sensor. CAUTION - The environment inside the sensor housing was completely desiccated and backfilled with pure argon prior to factory calibration. Opening the housing will introduce humidity (atmospheric water vapor) which will cause an immediate offset to the calibration and temporary drift instability.Swap the cable connections to the temperature and conductivity sensors to verify the operation of the acquisition circuit (SEASOFT permits the reversal of the channel assignments to permit the proper display of temperature or conductivity when this is done - use the SEACON program to do this).If no frequency indication occurs with the sensors swapped, disconnect the rubber molded plug from the sensor connector. +15 volts should be measured between the large pin and one of the small pins (if the large pin is up when looking into the plug, the +15 should be found on the left side small pin).The proper functioning of a frequency channel may be confirmed by connecting a frequency source (square or sine wave, 2 - 5 volts p-p) to right side small pin (as defined above) and thelarge (ground) pin of the sensor cable. The deck unit should read the frequency of the generator.The sensors may also be checked separately from the CTD by connecting a power source of 10 to 20 volts and observing the frequency output with an oscilloscope.Application Note No. 3 (in the 'app notes' section of this document) explains temperature/ conductivity sensor disassembly instructions. Look for broken leads or evidence of water leakage. 5-2.2 PRESSURE SENSORInternal pressure sensors are mounted inside foam insulation near the bottom of the card file. The red lead should be at +8 volts, the black lead at power common. The blue lead should be connected to Backplane via the small board that the sensor cabling is secured to. A properly operating sensor will exhibit a square wave frequency in the range 32 to 40 kHz at this point. For a discussion of possible fault conditions associated with this and subsequent acquisition circuitry, see Section 5-1.3 above.It is not possible to perform field repairs on a defective pressure sensor; any repair work must be done at the factory.The temperature compensation of the pressure makes use of a solid-state (bandgap) temperature sensor embedded in the pressure sensor. This element (Analog Devices type AD590) is a 2-terminal device which generates a current proportional to absolute temperature (1 microampere per °K). The current is input to an op amp on the Modulo 12P board; a current of opposite polarity is derived from the REF02 / Op215 reference and used as an offset source. The current difference is scaled through the 95K ohm precision fixed resistor R19 to create an input to the 12 bit A/D converter, U8. The A/D converter parallel output is strobed into the CD4021 shift registers in preparation for transmission to the deck unit.The white lead (from the pressure sensor) goes to -15 volts. The orange lead connects to pin 10 of the Modulo 12P board. Disconnect this lead and connect through a microammeter to power common. If the sensor is working correctly, the current should be approximately equal (in microamperes) to the ambient temperature in °K. If the temperature sensor appears to be ok, check the voltage levels associated with Modulo 12P board U3.5-2.3The dissolved oxygen sensorThe dissolved oxygen sensor should show a sensor current channel output of approximately +5 volts when the system is powered up. This 'saturation' condition will continue for up to aminute or two, after which the output will steadily decline until a stable reading (typically 2 volts) representative of atmospheric oxygen level is maintained.If the oxygen current channel voltage is zero upon power up, the sensor is defective. This condition can be caused by the sensor module proper (the small brown plastic element at the top of the DO sensor) breaking contact. See the Disassembly instructions in the 'dissolved oxygen' section which describe how the sense module's electrical contact is made.The electrical cable connecting the DO probe to the main CTD housing should be checked. Disconnect the cable from the probe and check for +15 volts between the large pin (common) and the left-hand pin (viewed from face with large pin up). Put a voltage source of approximately 5 volts between the large pin and the bottom small pin: the deck unit should read about 1024 (thumbwheel set the 3, left 4 digits of display). Connect to the right small pin: read 1024 on the right hand 4 digits.The DO probe housing may be disassembled to allow checking for broken wires, water leakage, etc1-3.1 CTD SENSORSThe temperature sensor (model SBE 3plus) is a compact module containing a pressure-protected high-speed thermistor and ‘Wein bridge oscillator’ interface electronics. The thermistor is the variable element in the Wein-bridge, while a precision Vishay resistor and two ultra-stable capacitors form the fixed components. The conductivity sensor (model SBE 4C) is similar in operation and configuration to the temperature sensor, except that the Wein-bridge variable element is the cell resistance. The Digiquartz pressure sensor also provides a variable frequency output. The sensor frequencies are measured using high-speed parallel counters and the resulting digital data in the form of count totals are transmitted serially to the SBE 11plus deck unit. The deck unit reconverts the count totals to numeric representations of the original frequencies.Sea-Bird conductivity and temperature sensors are calibrated by immersing them in a variable conductivity/temperature bath, while the pressure sensor is calibrated using a dead-weight pressure generator. The sensor output frequencies are tabulated along with the known physical input conditions of C, T, and P and the results used to obtain a series of calibration coefficients. The sensor frequency as output by the CTD deck unit is then the input to a conversion equationwhich - along with the original calibration coefficients - gives results in scientific units.Embedded in the pressure sensor is a semiconductor temperature sensor used to compensate the small ambient temperature sensitivity of the Digiquartz.. The calibration information for each sensor (C, T, and P) is contained in a series of numeric coefficients used in equations relating frequency to the measured parameter.SBE 9plus CTDs are shipped with SBE 3plus and SBE 4C sensors equipped with Sea-Bird's TC duct.This accessory, in addition to the SBE 5 pump provides uniform and constant flow of sea water past the temperature and conductivity sensors. The physical configuration of the duct causes the time interval between temperature and conductivity measurements to be known and constant. Knowledge of the time interval between measurements allows salinity calculations to be made with measurements from the same parcel of water.For a detailed discussion of the theory of operation of the SBE 4 (and by close analogy, the SBE 3plus), refer to the paper 'Development of a Small in-situ Conductivity Instrument' at the end of this manual.Application Note 38 describes the TC duct; refer to the 'app notes' section of this manual for more information. See also, individual specification sheets for SBE 3plus Premium CTD Temperature sensor and SBE 4 Conductivity sensor, also located at the end of this manual.作者:SEA-BIRD电子股份有限公司国籍:美国出处:SEA-BIRD电子股份有限公司故障诊断和维修(水下设备的问题)(译文1)当启动电源时在甲板单元和水下装置产生危及生命的高电压。