第3讲 DSP系统设计
DSP应用系统的设计共46页PPT资料
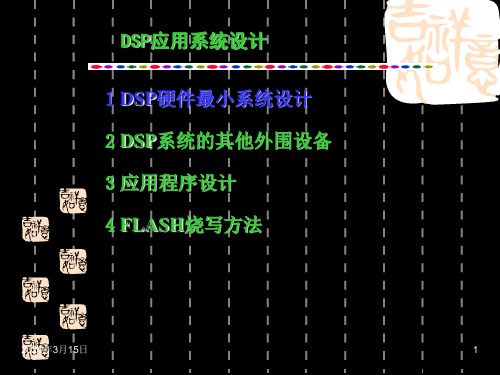
2020年3月15日
8
DSP应用系统设计
1 硬件设计概述
系统硬件设计过程:
第一步:确定硬件实现方案; 第二步:器件的选择; 第三步:原理图设计;
从第三步开始就进入系统的综合。 在原理图设计阶段必须清楚地了解器件 的特性、使用方法和系统的开发,必要 时可对单元电路进行功能仿真。
DSP芯片及DSP基本系统
程序和数据存储器
数/模和模/数转换器
模拟控制与处理路
各种控制口和通信口
电源处理电路和同步电路
2020年3月15日
2
DSP应用系统设计
1 硬件设计概述
一个典型的DSP目标板结构如下图。
信
防混叠 滤波器
ADC
号
•
•
预
• •
• •
处
理 、
防混叠 滤波器
ADC
MUX
、 程
平滑 滤波器
接口方式(串行还是并行)、工作电压(5V/3V)等来选择。
④ 逻辑控制器件的选择 系统的逻辑控制通常是用可编程逻辑器件来实现。 首先确定是采用CPLD还是FPGA; 其次根据自己的特长和公司芯片的特点选择哪家公 司的哪个系列的产品;
2020年3月15日最后还要根据DSP的频率来选择所使用的PLD器件。 6
DSP应用系统设计
1 DSP硬件最小系统设计 2 DSP系统的其他外围设备 3 应用程序设计 4 FLASH烧写方法
2020年3月15日
1
DSP应用系统设计
1 硬件系统设计概述
DSP系统的硬件设计又称为目标板设计,是在 考虑算法需求、成本、体积和功耗核算的基础上完 成的,一个典型的DSP目标板主要包括:
DSP应用系统设计实例教学设计
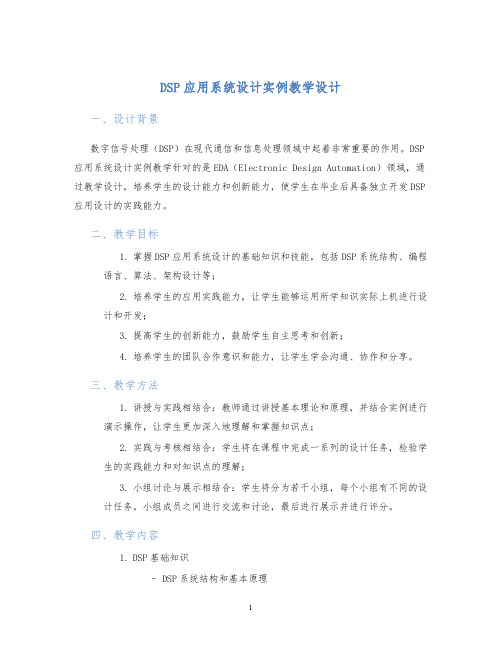
DSP应用系统设计实例教学设计一、设计背景数字信号处理(DSP)在现代通信和信息处理领域中起着非常重要的作用。
DSP 应用系统设计实例教学针对的是EDA(Electronic Design Automation)领域,通过教学设计,培养学生的设计能力和创新能力,使学生在毕业后具备独立开发DSP 应用设计的实践能力。
二、教学目标1.掌握DSP应用系统设计的基础知识和技能,包括DSP系统结构、编程语言、算法、架构设计等;2.培养学生的应用实践能力,让学生能够运用所学知识实际上机进行设计和开发;3.提高学生的创新能力,鼓励学生自主思考和创新;4.培养学生的团队合作意识和能力,让学生学会沟通、协作和分享。
三、教学方法1.讲授与实践相结合:教师通过讲授基本理论和原理,并结合实例进行演示操作,让学生更加深入地理解和掌握知识点;2.实践与考核相结合:学生将在课程中完成一系列的设计任务,检验学生的实践能力和对知识点的理解;3.小组讨论与展示相结合:学生将分为若干小组,每个小组有不同的设计任务,小组成员之间进行交流和讨论,最后进行展示并进行评分。
四、教学内容1.DSP基础知识–DSP系统结构和基本原理–DSP编程语言–DSP算法2.DSP应用开发–DSP应用需要的硬件和软件环境的搭建–DSP应用的开发流程3.DSP应用系统设计实例–声音信号的DSP处理–图像和视频信号的DSP处理–实时控制系统的DSP处理五、教学评估1.作业评估:根据学生提交的作业进行评估,重点考察学生实际应用能力;2.实验报告评估:针对学生完成的实验报告进行评估,重点考察学生对知识点的理解程度;3.课堂表现评估:通过学生的课堂出勤率和表现进行评估,重点考察学生的学习态度和团队合作能力;4.期末项目评估:最终项目是课程的重要评估指标,考察学生的实际应用能力、创新能力和团队协作能力。
六、教学成果通过这门课程,学生将掌握DSP应用系统设计的基础知识和技能,具备实际开发DSP应用设计的能力和独立思考的创新能力。
dsp课程设计设计方案
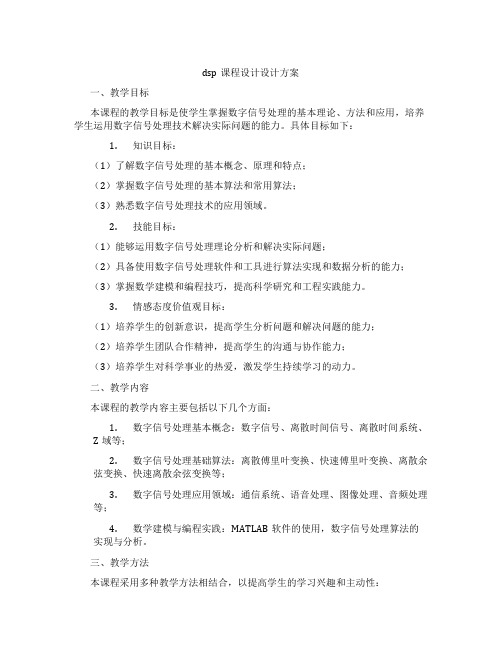
dsp课程设计设计方案一、教学目标本课程的教学目标是使学生掌握数字信号处理的基本理论、方法和应用,培养学生运用数字信号处理技术解决实际问题的能力。
具体目标如下:1.知识目标:(1)了解数字信号处理的基本概念、原理和特点;(2)掌握数字信号处理的基本算法和常用算法;(3)熟悉数字信号处理技术的应用领域。
2.技能目标:(1)能够运用数字信号处理理论分析和解决实际问题;(2)具备使用数字信号处理软件和工具进行算法实现和数据分析的能力;(3)掌握数学建模和编程技巧,提高科学研究和工程实践能力。
3.情感态度价值观目标:(1)培养学生的创新意识,提高学生分析问题和解决问题的能力;(2)培养学生团队合作精神,提高学生的沟通与协作能力;(3)培养学生对科学事业的热爱,激发学生持续学习的动力。
二、教学内容本课程的教学内容主要包括以下几个方面:1.数字信号处理基本概念:数字信号、离散时间信号、离散时间系统、Z域等;2.数字信号处理基础算法:离散傅里叶变换、快速傅里叶变换、离散余弦变换、快速离散余弦变换等;3.数字信号处理应用领域:通信系统、语音处理、图像处理、音频处理等;4.数学建模与编程实践:MATLAB软件的使用,数字信号处理算法的实现与分析。
三、教学方法本课程采用多种教学方法相结合,以提高学生的学习兴趣和主动性:1.讲授法:通过讲解基本概念、原理和算法,使学生掌握数字信号处理的基本知识;2.讨论法:学生进行课堂讨论,培养学生的思考能力和团队协作能力;3.案例分析法:分析实际案例,使学生了解数字信号处理在工程应用中的重要性;4.实验法:通过实验操作,让学生亲手实践,加深对数字信号处理算法的理解和掌握。
四、教学资源本课程的教学资源包括以下几个方面:1.教材:选用国内外优秀教材,如《数字信号处理》(郑志中)、《数字信号处理原理与应用》(李翠莲)等;2.参考书:提供相关领域的参考书籍,如《数字信号处理教程》(谢维信)、《数字信号处理学习指导》(张刺激)等;3.多媒体资料:制作课件、教学视频等,以图文并茂的形式展示教学内容;4.实验设备:配备计算机、MATLAB软件、信号发生器、示波器等实验设备,为学生提供实践操作的机会。
DSP课程设计
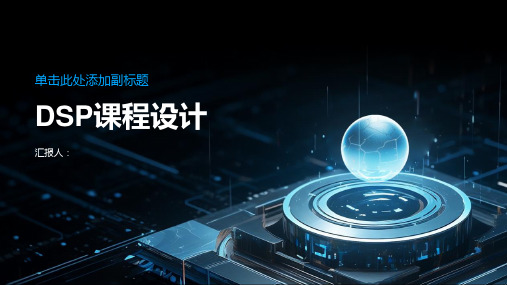
压缩:对数字信号进行压 缩处理,如MP3、JPEG等
数字信号处理:对数字信 号进行各种处理,如滤波、
变换、压缩等
采样:将连续时间信号离 散化,得到数字信号
编码:将数字信号转换为 适合传输或存储的格式
变换:对数字信号进行变 换处理,如FFT、DCT等
数字信号处理算法的分类和特点
线性和非线性算法:线性算法简单易实现,非线性算法处理能力强 时域和频域算法:时域算法直观,频域算法处理速度快 确定性和随机性算法:确定性算法稳定性好,随机性算法适应性强 数字滤波器:包括FIR和IIR滤波器,FIR滤波器线性相位,IIR滤波器非线性相位 数字信号处理算法特点:速度快、精度高、灵活性强、易于实现复杂算法
感谢观看
汇报人:
开发环境:CCS、IAR等用于开发DSP程序
03
DSP系统设计
数字信号处理系统的基本组成和原理
• 数字信号处理器(DSP):负责处理数字信号,实现各种信号处理算法 • 存储器:存储程序和数据,包括RAM和ROM • 输入/输出设备:接收和输出信号,如ADC、DAC、UART等 • 电源:为系统提供稳定的电源电压 • 控制单元:控制整个系统的运行,包括中断、定时器等 • 总线:连接各个部件,实现数据传输和通信 • 软件:实现各种信号处理算法,如FFT、FIR、IIR等 • 硬件:实现各种信号处理功能,如ADC、DAC、FIFO等 • 数字信号处理系统的基本原理:通过数字信号处理器(DSP)实现各种信号处理算法,如FFT、FIR、
数字信号处理算法的实现实例和演示
快速傅里叶变换 (FFT):用于信 号频谱分析,实现 快速计算
自适应滤波器:根 据输入信号自动调 整滤波器参数,实 现信号处理
数字滤波器:用于 信号滤波,实现信 号处理
dsp的课程设计
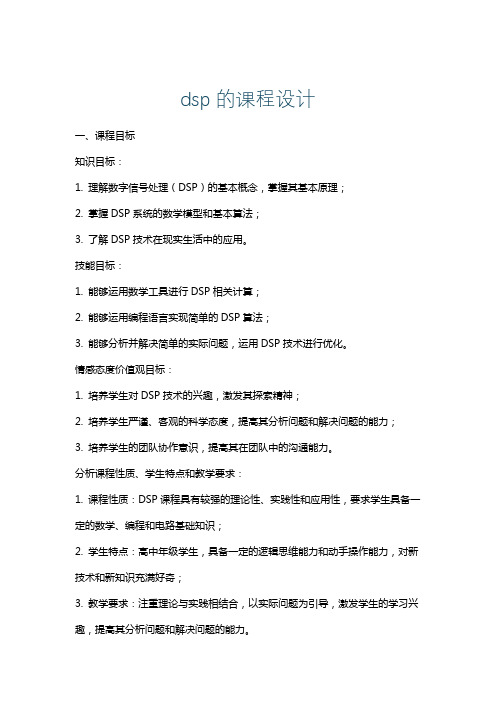
dsp的课程设计一、课程目标知识目标:1. 理解数字信号处理(DSP)的基本概念,掌握其基本原理;2. 掌握DSP系统的数学模型和基本算法;3. 了解DSP技术在现实生活中的应用。
技能目标:1. 能够运用数学工具进行DSP相关计算;2. 能够运用编程语言实现简单的DSP算法;3. 能够分析并解决简单的实际问题,运用DSP技术进行优化。
情感态度价值观目标:1. 培养学生对DSP技术的兴趣,激发其探索精神;2. 培养学生严谨、客观的科学态度,提高其分析问题和解决问题的能力;3. 培养学生的团队协作意识,提高其在团队中的沟通能力。
分析课程性质、学生特点和教学要求:1. 课程性质:DSP课程具有较强的理论性、实践性和应用性,要求学生具备一定的数学、编程和电路基础知识;2. 学生特点:高中年级学生,具备一定的逻辑思维能力和动手操作能力,对新技术和新知识充满好奇;3. 教学要求:注重理论与实践相结合,以实际问题为引导,激发学生的学习兴趣,提高其分析问题和解决问题的能力。
课程目标分解:1. 知识目标:通过本课程的学习,使学生掌握DSP的基本概念、原理和算法;2. 技能目标:通过实践操作,使学生能够运用数学工具和编程语言实现DSP 算法;3. 情感态度价值观目标:通过团队合作和实际问题解决,培养学生对DSP技术的兴趣,提高其科学素养和团队协作能力。
二、教学内容1. 数字信号处理基本概念:信号的定义、分类及特性;离散时间信号与系统;傅里叶变换及其性质。
2. DSP数学基础:复数运算;欧拉公式;离散傅里叶变换(DFT)及其快速算法(FFT)。
3. 数字滤波器设计:滤波器类型;无限长冲击响应(IIR)滤波器和有限长冲击响应(FIR)滤波器设计方法;滤波器的实现与优化。
4. DSP算法实现:快速傅里叶变换(FFT)算法;数字滤波器算法;数字信号处理中的数学优化方法。
5. DSP应用案例分析:语音信号处理;图像信号处理;通信系统中的应用。
DSP系统的设计过程
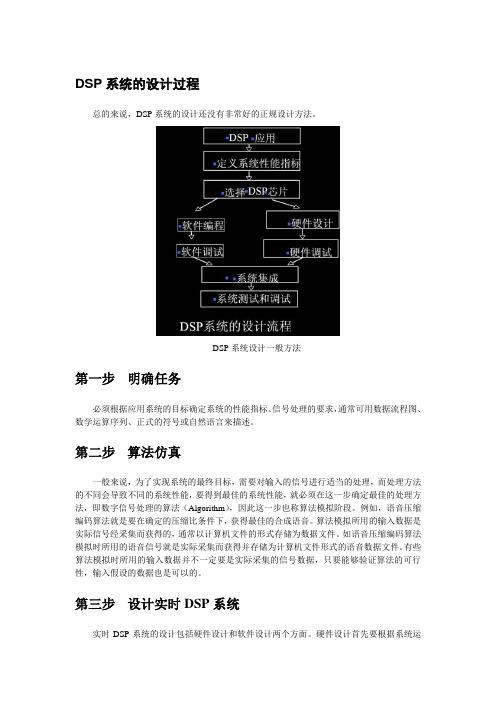
DSP系统的设计过程总的来说,DSP系统的设计还没有非常好的正规设计方法。
DSP系统设计一般方法第一步明确任务必须根据应用系统的目标确定系统的性能指标、信号处理的要求,通常可用数据流程图、数学运算序列、正式的符号或自然语言来描述。
第二步算法仿真一般来说,为了实现系统的最终目标,需要对输入的信号进行适当的处理,而处理方法的不同会导致不同的系统性能,要得到最佳的系统性能,就必须在这一步确定最佳的处理方法,即数字信号处理的算法(Algorithm),因此这一步也称算法模拟阶段。
例如,语音压缩编码算法就是要在确定的压缩比条件下,获得最佳的合成语音。
算法模拟所用的输入数据是实际信号经采集而获得的,通常以计算机文件的形式存储为数据文件。
如语音压缩编码算法模拟时所用的语音信号就是实际采集而获得并存储为计算机文件形式的语音数据文件。
有些算法模拟时所用的输入数据并不一定要是实际采集的信号数据,只要能够验证算法的可行性,输入假设的数据也是可以的。
第三步设计实时DSP系统实时DSP系统的设计包括硬件设计和软件设计两个方面。
硬件设计首先要根据系统运算量的大小、对运算精度的要求、系统成本限制以及体积、功耗等要求选择合适的DSP芯片。
然后设计DSP芯片的外围电路及其他电路。
软件设计和编程主要根据系统要求和所选的DSP芯片编写相应的DSP汇编程序,若系统运算量不大且有高级语言编译器支持,也可用高级语言(如C语言)编程。
由于现有的高级语言编译器的效率还比不上手工编写汇编语言的效率,因此在实际应用系统中常常采用高级语言和汇编语言的混合编程方法,即在算法运算量大的地方,用手工编写的方法编写汇编语言,而运算量不大的地方则采用高级语言。
采用这种方法,既可缩短软件开发的周期,提高程序的可读性和可移植性,又能满足系统实时运算的要求。
DSP硬件和软件设计完成后,就需要进行硬件和软件的调试。
软件的调试一般借助于DSP开发工具,如软件模拟器、DSP开发系统或仿真器等。
《DSP应用系统设计》课件
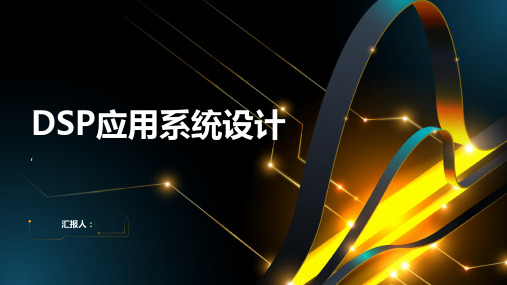
DSP应用系统广泛应用于通信、多媒体、 工业控制等领域。
DSP应用系统的设计需要考虑到性能、成 本、功耗等多个因素。
DSP应用系统的特点
高性能:处理速度快,计 算能力强
灵活性:可编程,可定制, 可扩展
实时性:实时处理数据, 响应速度快
稳定性:可靠性高,抗干 扰能力强
应用广泛:应用于通信、 信号处理、图像处理等领 域
01 02
03 04
05
DSP芯片的分类:根据应用领域和功能,可以分为通用型DSP芯片、 专用型DSP芯片和混合型DSP芯片。
通用型DSP芯片:具有较高的灵活性和可编程性,适用于多种应用 领域。
专用型DSP芯片:针对特定应用领域进行优化设计,具有较高的性 能和效率。
混合型DSP芯片:结合了通用型和专用型DSP芯片的优点,具有较 高的灵活性和性能。
常用数字信号处理算法
快速傅里叶变换(FFT):用于信号频谱分析 离散小波变换(DWT):用于信号去噪和压缩 自适应滤波器:用于信号处理和系统辨识 卡尔曼滤波器:用于信号估计和预测 神经网络:用于模式识别和预测 遗传算法:用于优化问题和参数估计
算法设计流程与优化
确定算法目标:明确算法需要解决的问题和性能要求
应用实例:包括图像处理、信号处 理、语音识别等
调试与测试方法
单元测试:对每个模块进行独立测试,确保其功能正确
集成测试:将各个模块集成在一起进行测试,确保系统整体功能正确
压力测试:对系统进行高负载测试,确保系统在高负载情况下仍能正常 运行
性能测试:对系统的性能进行测试,确保系统满足性能要求
稳定性测试:对系统进行长时间运行测试,确保系统在长时间运行下仍 能保持稳定
硬件设计需要遵循一定的规 范和标准,如IEEE、ISO等
DSP课程设计控制系统
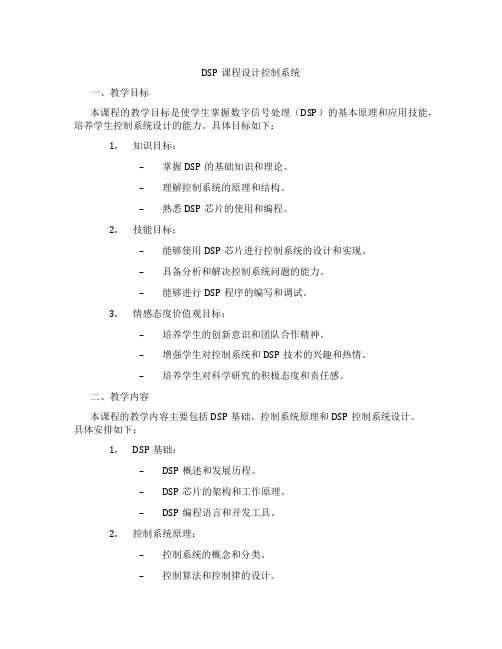
DSP课程设计控制系统一、教学目标本课程的教学目标是使学生掌握数字信号处理(DSP)的基本原理和应用技能,培养学生控制系统设计的能力。
具体目标如下:1.知识目标:–掌握DSP的基础知识和理论。
–理解控制系统的原理和结构。
–熟悉DSP芯片的使用和编程。
2.技能目标:–能够使用DSP芯片进行控制系统的设计和实现。
–具备分析和解决控制系统问题的能力。
–能够进行DSP程序的编写和调试。
3.情感态度价值观目标:–培养学生的创新意识和团队合作精神。
–增强学生对控制系统和DSP技术的兴趣和热情。
–培养学生对科学研究的积极态度和责任感。
二、教学内容本课程的教学内容主要包括DSP基础、控制系统原理和DSP控制系统设计。
具体安排如下:1.DSP基础:–DSP概述和发展历程。
–DSP芯片的架构和工作原理。
–DSP编程语言和开发工具。
2.控制系统原理:–控制系统的概念和分类。
–控制算法和控制律的设计。
–系统稳定性和性能分析。
3.DSP控制系统设计:–DSP控制系统的结构和组成。
–控制系统的设计方法和步骤。
–DSP控制程序的编写和调试。
三、教学方法为了激发学生的学习兴趣和主动性,本课程将采用多种教学方法,包括:1.讲授法:通过教师的讲解,使学生掌握DSP基础知识和控制系统原理。
2.案例分析法:分析实际案例,使学生了解DSP控制系统的应用和设计方法。
3.实验法:进行DSP控制系统的实验,培养学生的动手能力和实践能力。
4.讨论法:学生进行分组讨论,促进学生之间的交流和合作。
四、教学资源为了支持教学内容和教学方法的实施,我们将准备以下教学资源:1.教材:选用权威、实用的DSP和控制系统教材,为学生提供系统性的学习资料。
2.参考书:提供相关的参考书籍,丰富学生的知识储备。
3.多媒体资料:制作精美的课件和教学视频,增强课堂教学的趣味性和效果。
4.实验设备:准备DSP开发板和相关的实验设备,为学生提供实践操作的机会。
五、教学评估本课程的评估方式包括平时表现、作业和考试等。
dsp课程设计
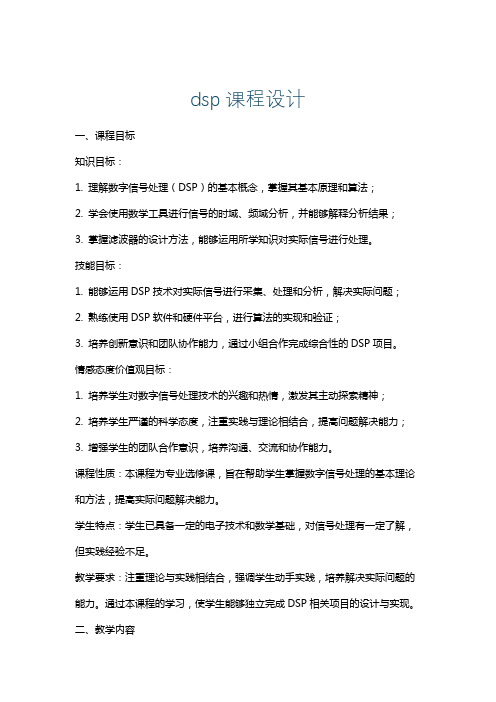
dsp课程设计一、课程目标知识目标:1. 理解数字信号处理(DSP)的基本概念,掌握其基本原理和算法;2. 学会使用数学工具进行信号的时域、频域分析,并能够解释分析结果;3. 掌握滤波器的设计方法,能够运用所学知识对实际信号进行处理。
技能目标:1. 能够运用DSP技术对实际信号进行采集、处理和分析,解决实际问题;2. 熟练使用DSP软件和硬件平台,进行算法的实现和验证;3. 培养创新意识和团队协作能力,通过小组合作完成综合性的DSP项目。
情感态度价值观目标:1. 培养学生对数字信号处理技术的兴趣和热情,激发其主动探索精神;2. 培养学生严谨的科学态度,注重实践与理论相结合,提高问题解决能力;3. 增强学生的团队合作意识,培养沟通、交流和协作能力。
课程性质:本课程为专业选修课,旨在帮助学生掌握数字信号处理的基本理论和方法,提高实际问题解决能力。
学生特点:学生已具备一定的电子技术和数学基础,对信号处理有一定了解,但实践经验不足。
教学要求:注重理论与实践相结合,强调学生动手实践,培养解决实际问题的能力。
通过本课程的学习,使学生能够独立完成DSP相关项目的设计与实现。
二、教学内容1. 数字信号处理基础:信号与系统、离散时间信号与系统、线性时不变系统、卷积运算等;2. 离散傅里叶变换:傅里叶级数、离散傅里叶变换(DFT)、快速傅里叶变换(FFT)等;3. 数字滤波器设计:滤波器原理、无限长冲激响应(IIR)滤波器设计、有限长冲激响应(FIR)滤波器设计等;4. 数字信号处理应用:数字信号处理在语音、图像、通信等领域的应用案例分析;5. 实践教学:使用DSP软件和硬件平台进行算法实现和验证,开展综合性的DSP项目。
教学大纲安排:第一周:数字信号处理基础第二周:离散时间信号与系统第三周:线性时不变系统与卷积运算第四周:离散傅里叶变换第五周:快速傅里叶变换第六周:数字滤波器设计原理第七周:IIR滤波器设计第八周:FIR滤波器设计第九周:数字信号处理应用案例分析第十周:实践教学与项目开展教学内容与教材关联性:本课程教学内容依据教材章节进行安排,涵盖数字信号处理的基本理论、方法和应用,确保学生系统掌握DSP相关知识。
DSP应用课程设计课件 第3讲 利用DSP实现信号谱分析

13
北京交通大学 国家工科电工电子教学基地
DSP应用课程设计
CMD文件
MEMORY { PAGE 0: VECS: origin = 0080h, length = 0080h PRAM: origin = 7600h, length = 8000h PAGE 1: STACK: origin = 1180h, length = 0560h EXRAM: origin = 1ff1h, length = 4000h } SECTIONS { .cinit > PRAM PAGE 0 .text > PRAM PAGE 0 .vectors > VECS PAGE 0
利用DSP实现信号谱分析
一、实验目的 二、实验原理 三、实验内容
1
北京交通大学 国家工科电工电子教学基地
DSP应用课程设计
一、实验目的 1.掌握FFT的算法原理和基本性质
2.掌握利用Matlab产生数据的方法
3.掌握调用TMS320C54x DSPLIB库函数的方法
4.掌握CCS基本调试技术
特殊要求: 输入数组首地址最低log2(2*n)为必须为0
12
北京交通大学 国家工科电工电子教学基地
DSP应用课程设计
cbrev()和rfft()使用的例
#define NX DATA y[NX]; 16
DATA x[NX];
cbrev(x,y,NX/2);
rfft(y,NX,1);
一个N点序列x[k]的DFT为X[m],以及IDFT分别定义为:
km X [m] x[k ]WN , m 0,1,, N 1 k 0 N 1
1 x[k ] N
N 1
DSP设计课程设计

DSP设计课程设计一、课程目标知识目标:1. 让学生理解DSP(数字信号处理)的基本原理和概念,包括采样定理、傅里叶变换和数字滤波器设计等。
2. 使学生掌握DSP算法的数学推导和实现方法,具备使用DSP芯片进行信号处理的能力。
3. 帮助学生了解DSP技术在通信、音视频处理等领域的应用。
技能目标:1. 培养学生运用数学工具进行DSP相关算法推导和仿真能力。
2. 提高学生实际操作DSP芯片,完成信号处理实验的能力。
3. 培养学生团队协作和沟通能力,能够就DSP技术问题进行讨论和分析。
情感态度价值观目标:1. 激发学生对数字信号处理技术的兴趣,培养其探索精神和创新意识。
2. 培养学生严谨的科学态度,注重实践和理论相结合。
3. 引导学生关注DSP技术在国家和社会发展中的应用,增强其社会责任感和使命感。
分析课程性质、学生特点和教学要求,本课程旨在让学生通过学习DSP设计,掌握数字信号处理的基本原理和方法,培养其实践操作能力。
课程目标分解为具体学习成果,以便后续教学设计和评估:学生能够独立完成DSP算法推导、仿真和实验操作,具备解决实际问题的能力,并在团队合作中发挥积极作用。
二、教学内容1. DSP基本原理与概念- 采样定理与信号重建- 傅里叶变换及其应用- 数字滤波器设计原理2. DSP算法及其数学推导- 离散时间信号处理基础- 快速傅里叶变换(FFT)算法- 数字滤波器算法实现3. DSP芯片与应用- DSP芯片架构与特点- DSP芯片编程与实验操作- DSP技术在通信、音视频处理等领域的应用案例4. 教学大纲安排与进度- 第一阶段:基本原理与概念(2周)- 课本章节:第1-3章- 第二阶段:DSP算法及其数学推导(3周)- 课本章节:第4-6章- 第三阶段:DSP芯片与应用(3周)- 课本章节:第7-9章教学内容按照课程目标进行选择和组织,确保科学性和系统性。
在教学过程中,教师将依据教学大纲,引导学生学习课本相关章节,完成教学内容的学习。
dsp课程设计图文

dsp课程设计图文一、教学目标本课程的教学目标是使学生掌握DSP(数字信号处理器)的基本原理、应用和编程方法。
通过本课程的学习,学生将能够:1.理解DSP的基本概念、结构和分类;2.掌握DSP的基本算法和编程技巧;3.熟悉DSP的开发工具和仿真环境;4.能够运用DSP解决实际信号处理问题。
二、教学内容本课程的教学内容主要包括以下几个部分:1.DSP的基本原理:DSP的概念、发展历程、分类和应用领域;2.DSP的结构与工作原理:哈佛结构、冯·诺依曼结构、DSP的内部组成和信号流程;3.DSP的基本算法:数字滤波器、快速傅里叶变换、自适应滤波器等;4.DSP的编程方法:C语言编程、汇编语言编程、算法实现和代码优化;5.DSP的开发工具和仿真环境:CCS、MATLAB等工具的使用。
三、教学方法为了提高学生的学习兴趣和主动性,本课程将采用多种教学方法:1.讲授法:讲解DSP的基本原理、结构和算法;2.讨论法:学生讨论DSP的应用案例和编程技巧;3.案例分析法:分析实际信号处理问题,引导学生运用DSP解决问题;4.实验法:让学生动手实践,熟悉DSP的开发工具和仿真环境。
四、教学资源为了支持教学内容和教学方法的实施,本课程将准备以下教学资源:1.教材:选用权威、实用的DSP教材,为学生提供系统的理论知识;2.参考书:提供相关的DSP参考书籍,方便学生深入研究;3.多媒体资料:制作课件、视频等多媒体资料,丰富学生的学习体验;4.实验设备:准备DSP开发板和仿真器,让学生进行实践操作。
五、教学评估本课程的评估方式包括平时表现、作业、考试等,以全面反映学生的学习成果。
具体评估方式如下:1.平时表现:包括课堂参与度、提问回答、小组讨论等,占总分的30%;2.作业:布置适量的作业,巩固所学知识,占总分的20%;3.考试:包括期中考试和期末考试,期中考试占总分的20%,期末考试占总分的30%。
六、教学安排本课程的教学安排如下:1.教学进度:按照教材的章节顺序进行教学,确保学生系统地掌握DSP知识;2.教学时间:每周安排2课时,共16周,确保在有限的时间内完成教学任务;3.教学地点:教室和实验室,以便进行理论讲解和实践操作。
DSP 第3讲 中断系统

§3.11 中断和中断向量表
中断号(K) 优先级 名 称 向量位置 功 能
16
17 18 19 20 21 22
3
4 5 6 7 8 9
INT0/SINT0
INT1/SINT1 IN12/SINT2 TINT0/SINT3 BRINT0/SINT4 BXINT0/SINT5 DMAC0/SINT6
§3.12 VC5402的中断向量表文件—续
RESET: ;复位中断,优先级最高 BD _c_int00 ;执行完该语句下面的一条语句后,跳转到_c_int00处 STM #128,SP ;设置堆栈大小为128个字 nmi:RETE ;nmi非屏蔽中断返回,下面依次为VC5402的各个中断, NOP ;每个只占4个字节 NOP NOP sint17 .space 4*16 ;VC5402的软中断 sint18 .space 4*16 ;.space表示开辟空间(单位:bit) sint19 .space 4*16 sint20 .space 4*16 sire21 .space 4*16 sint22 .space 4*16 sint23 .space 4*16 sint24 .space 4*16 sint25 .space 4*16 sint26 .space 4*16 sint27 .space 4*16 sint28 .space 4*16 sint29 .space 4*16 sint30 .space 4*16 ;这部分可以直接写为 .space 14*4*16
(3)IMR屏蔽位为1。(使能一个中断)
§3.6 执行中断服务程序(ISR)
当应答中断后,CPU会采取如下的操作:
dsp项目课程设计
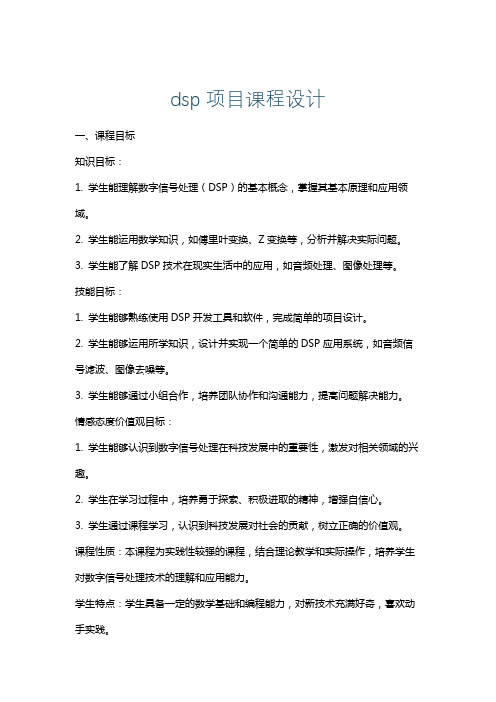
dsp项目课程设计一、课程目标知识目标:1. 学生能理解数字信号处理(DSP)的基本概念,掌握其基本原理和应用领域。
2. 学生能运用数学知识,如傅里叶变换、Z变换等,分析并解决实际问题。
3. 学生能了解DSP技术在现实生活中的应用,如音频处理、图像处理等。
技能目标:1. 学生能够熟练使用DSP开发工具和软件,完成简单的项目设计。
2. 学生能够运用所学知识,设计并实现一个简单的DSP应用系统,如音频信号滤波、图像去噪等。
3. 学生能够通过小组合作,培养团队协作和沟通能力,提高问题解决能力。
情感态度价值观目标:1. 学生能够认识到数字信号处理在科技发展中的重要性,激发对相关领域的兴趣。
2. 学生在学习过程中,培养勇于探索、积极进取的精神,增强自信心。
3. 学生通过课程学习,认识到科技发展对社会的贡献,树立正确的价值观。
课程性质:本课程为实践性较强的课程,结合理论教学和实际操作,培养学生对数字信号处理技术的理解和应用能力。
学生特点:学生具备一定的数学基础和编程能力,对新技术充满好奇,喜欢动手实践。
教学要求:教师需结合课本内容,以实际项目为导向,引导学生掌握基本理论,提高实际操作能力。
在教学过程中,注重培养学生的团队协作和创新能力,提高学生的综合素质。
通过课程目标分解,确保学生能够达到预期学习成果,为后续教学设计和评估提供依据。
二、教学内容1. 数字信号处理基础理论:- 傅里叶变换理论及其应用- Z变换及其性质- 离散时间信号与系统2. DSP算法与应用:- 数字滤波器设计- 快速傅里叶变换(FFT)算法- 数字信号处理在音频、图像领域的应用3. DSP实践项目:- 项目一:音频信号处理(滤波、增强)- 项目二:图像处理(去噪、边缘检测)- 项目三:DSP综合应用(如语音识别、图像识别)4. 教学内容的安排与进度:- 基础理论部分:占总课时的1/3,以课本相关章节为基础,逐步引导学生掌握基本概念和原理。
DSP系统设计
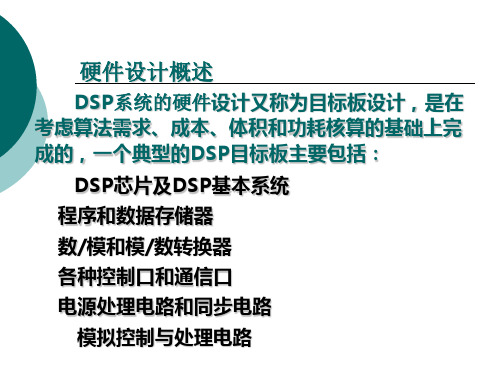
① 时钟方式寄存器CLKMD 时钟方式寄存器CLKMD 用来定义PLL时钟模块中的时钟配置, PLL时钟模块中的时钟配置 用来定义PLL时钟模块中的时钟配置,为用户提 供各种时钟乘系数,并能直接通断PLL PLL。 供各种时钟乘系数,并能直接通断PLL。
15 ~ 12 11 10 ~ 3 2 1 0
DVDD CVDD
3.电源电压的产生 3.电源电压的产生
第一种方案: 第一种方案:
5V
电压调节器1 电压调节器1
DVDD (3.3V) CVDD (1.8V)
5V电源通过两个电压 5V电源通过两个电压 调节器,分别产生3 调节器,分别产生3.3V和 1.8V电压。 电压。
电压调节器2 电压调节器2
系统硬件设计过程: 系统硬件设计过程: 第一步:确定硬件实现方案; 第一步:确定硬件实现方案; 第二步:器件的选择; 第二步:器件的选择; 第三步:原理图设计; 第三步:原理图设计; 第四步:PCB设计 设计; 第四步:PCB设计; 第五步:硬件调试; 第五步:硬件调试;
一、电源电路的设计
1.电源电压和电流要求 1.电源电压和电流要求 为了获得更好的电源性能, C54x采用双电源 为了获得更好的电源性能,’C54x采用双电源 供电方式。 供电方式。 内核电源CV 采用1 内核电源CVDD:采用1.8V。 主要为芯片的内部逻辑提供电压。 主要为芯片的内部逻辑提供电压。 包括CPU 时钟电路和所有的外设逻辑。 CPU、 包括CPU、时钟电路和所有的外设逻辑。 I/O电源 电源DV 采用3 I/O电源DVDD:采用3.3V。 主要供I/O接口使用。 I/O接口使用 主要供I/O接口使用。 可直接与外部低压器件接口, 可直接与外部低压器件接口,而无需额外的电 平变换电路。 平变换电路。
dsp语音通信系统课程设计

dsp语音通信系统课程设计一、课程目标知识目标:1. 理解数字信号处理(DSP)的基本原理,掌握其在语音通信中的应用。
2. 学习并掌握语音信号的采集、处理、传输和接收等基本环节。
3. 掌握语音信号的数字化过程,包括采样、量化、编码等关键技术。
技能目标:1. 能够运用所学知识,设计并实现一个简单的DSP语音通信系统。
2. 培养学生动手实践能力,学会使用相关软件和硬件工具进行语音信号处理和通信。
3. 提高学生的问题分析和解决能力,能够针对实际通信过程中的问题进行优化和调试。
情感态度价值观目标:1. 培养学生对通信工程领域的兴趣,激发学生的创新意识和探索精神。
2. 培养学生的团队合作意识,学会与他人合作共同解决问题。
3. 增强学生的责任心和使命感,认识到通信技术在我国经济社会发展中的重要地位。
分析课程性质、学生特点和教学要求:1. 课程性质:本课程为电子信息类学科的专业课程,具有较强的理论性和实践性。
2. 学生特点:学生已具备一定的电子技术和数字信号处理基础,具有一定的编程和实践能力。
3. 教学要求:结合实际应用,注重理论与实践相结合,强调学生的动手实践能力和创新能力。
二、教学内容1. DSP基本原理回顾:包括数字信号处理的基本概念、系统函数、傅里叶变换等基础理论。
相关教材章节:第一章 数字信号处理基础2. 语音信号处理技术:学习语音信号的特性、预处理方法、特征提取等关键技术。
相关教材章节:第二章 语音信号处理技术3. 语音信号的数字化:介绍语音信号的采样、量化、编码等过程,分析其影响通信质量的因素。
相关教材章节:第三章 语音信号的数字化4. 语音通信系统设计:学习语音通信系统的基本架构,探讨各个环节的设计方法。
相关教材章节:第四章 语音通信系统设计5. DSP语音通信系统实践:结合实际案例,指导学生设计并实现一个简单的DSP语音通信系统。
相关教材章节:第五章 实践环节6. 系统优化与调试:分析通信过程中的问题,探讨优化和调试方法,提高通信质量。
dsp最小系统设计课程设计

dsp最小系统设计课程设计一、课程目标知识目标:1. 理解DSP最小系统设计的原理和概念,掌握其主要组成部分及功能;2. 学会分析DSP最小系统的性能指标,了解不同设计参数对系统性能的影响;3. 掌握DSP最小系统的硬件设计和软件编程方法,能够实现基本的信号处理功能。
技能目标:1. 培养学生运用所学知识进行DSP最小系统硬件选型、电路设计和调试的能力;2. 培养学生编写DSP程序,实现信号处理算法的能力;3. 培养学生运用仿真软件对DSP最小系统进行性能分析和优化的能力。
情感态度价值观目标:1. 培养学生热爱科学,积极探索和实践的精神,增强其对电子信息和信号处理领域的兴趣;2. 培养学生具备良好的团队协作精神,学会与他人合作解决问题;3. 培养学生严谨、细致、负责的工作态度,养成良好地工程素养。
课程性质:本课程为电子信息类专业高年级学生的专业实践课程,旨在培养学生的实际工程能力和创新意识。
学生特点:学生已具备一定的电子技术、信号处理和编程基础,具有较强的学习能力和动手能力。
教学要求:结合课程性质和学生特点,通过本课程的学习,使学生能够独立完成DSP最小系统的设计与实现,为今后从事电子信息领域工作打下坚实基础。
在教学过程中,注重理论与实践相结合,鼓励学生创新和探索。
将课程目标分解为具体的学习成果,便于教学设计和评估。
二、教学内容1. DSP最小系统基本原理:介绍DSP芯片的工作原理、性能特点及最小系统的基本构成,关联课本第3章内容。
- DSP芯片选型与性能参数- 最小系统构成及功能模块2. DSP最小系统硬件设计:讲解硬件设计方法、电路原理及硬件调试技巧,关联课本第4章内容。
- 硬件设计流程与关键参数- 电路原理图绘制与PCB设计- 硬件调试与测试方法3. DSP最小系统软件编程:介绍软件编程方法、算法实现及程序调试技巧,关联课本第5章内容。
- 汇编语言与C语言编程- 常用算法的DSP实现- 程序调试与优化方法4. DSP最小系统性能分析与优化:分析系统性能指标,探讨优化策略,关联课本第6章内容。
DSP系统设计

Designing a DSPSystemDoc #1.0Application NoteDigital Signalprocessing TechnologyNorbert Nölker & Adolf Klemenz GbRGelderner Straße 36D - 47647 Kerkenphone+49 (0) 2833 / 570 977fax+49 (0) 2833 / 33 28email info@dsignt.dewww http://www.dsignt.deDesigning a DSP System Revision History1.0 August 1999 - initial release© D.SignT 1999 Doc #1.011 INTRODUCTION (4)2 DATA ACQUISITION (5)2.1 Serial Interface (5)2.2 Parallel Interface (7)2.3 Which maximum Data Acquisition Rate is possible ? (8)2.3.1 Interrupts (8)2.3.2 Busy-Polling (11)2.3.3 DMA (13)2.3.4 Still too slow ? (15)3 DATA OUTPUT (17)4 CONTROL INTERFACE (18)4.1 Host Interface (18)4.2 Asynchronous Communication (19)4.3 Bit-programmable I/O Ports (19)4.4 Sensors (20)4.5 Networks (20)4.6 Other I/O Interfaces (20)4.7 Control Software (21)5 DSP AND MEMORY (23)5.1 Memory Organisation (23)5.2 Operational Units (24)5.3 Data Format (26)5.4 Arithmetic (27)5.5 Built-in Peripherals (27)5.6 Benchmarks (28)© D.SignT 1999 Doc #1.026 COMPILER AND CODE DEVELOPMENT (30)6.1 Assembly Language Modules (31)6.2 The Influence of the Linker on Execution Performance (31)6.3 Real-Time Operating Systems (32)6.4 Debugging (33)7 CONCLUSION (34)© D.SignT 1999 Doc #1.031 IntroductionDSPs provide unbeatable performance in processing real-world data. Architecture is optimised to sustain high transfer rates and single-cycle execution of the fun-damental signal processing operations. No other processors exist which offer these performance levels at comparable system cost and power requirements.Signal processing algorithms are the dominating parts of most DSP applications, however lots of secondary tasks have to be executed:• Data must be acquired and/or output• Signal processing is controlled by environmental conditions and user settings, thus communication with host systems, user interfaces or sensors is required.• The results of signal processing must be evaluated and transmitted to other systems for further processing or visualisation or they are used to control ac-tors and machinery.The impact these secondary tasks have on signal processing execution time is often neglected. If you take a look at typical benchmark code provided by the DSP manufacturers, you will find data readily available in the DSP memory and often all data busses fully occupied by the signal processing data movements. This is far away from reality: In parallel with signal processing, you will have to read A/D converters and store the samples in an acquisition buffer, sample I/O ports, and read or write host controllers or communication devices. These tasks will often cause interrupts, bus locks or bus collisions.The subject of this application note is to describe typical interface techniques for data acquisition and control, the associated software, and discussion of the influence on DSP operation speed. We will try to clarify dependencies and pecu-liarities of the different DSP architectures which are not apparent after a first glance at the data sheets. Most areas from data acquisition via control tasks up to code generation will be covered. We will however not discuss typical signal processing algorithms as there is lots of excellent literature available about it, instead we will try to draw your attention to the interaction of signal processing and framework tasks.© D.SignT 1999 Doc #1.042 Data AcquisitionMost DSP systems will be used to process some kind of real-world data, either acquired by A/D converters directly connected to the DSP systems or transmitted digitally via point-to-point links or networks.2.1 Serial InterfaceThe first choice to connect the incoming data stream to the DSP is the synchro-nous serial port, a built-in peripheral on all general purpose DSPs. Benefits of the serial interface are not only a reduced pin count and less glue-logic, the main advantage is independence from external bus operations. The serial interface operates completely 'on it's own' , whereas data acquisition via a parallel bus interface may conflict with other bus transactions.A serial interface is typically 'calmer' than a parallel interface, no interference from other bus transactions is radiated to the data acquisition devices, and it is easier to de-couple and isolate.Figure 2-1 Serial InterfaceAll general purpose DSPs offer at least one serial interface which can be config-ured via control registers to meet the peripheral's requirements. Polarity, direc-© D.SignT 1999 Doc #1.05tion, frame sync type and timing, and data format can be adjusted. Industry stan-dard converters and Codecs can be connected without additional glue logic. You will often find the serial port configuration settings for popular DSPs in the con-verter's application notes.Many DSPs offer a multi-channel support for time division multiplex interfaces, like the T1/E1 format. Only pre-selected timeslots will be read to reduce data transfer and relieve the DSP core.Clocks and frame synchronisation can be generated internally, derived from the DSP system clock, or provided externally, i.e. the serial interface can be operated in master and slave mode. The maximum clock frequency of the serial port limits this interface technique to sampling frequencies below 500 kHz, yielding in a serial clock frequency of 8 MHz for a 16 Bit / 500 KSPS converter. Although modern DSPs are capable of much higher serial clocks, serial interface convert-ers with higher sampling rates are rare. You may however consider to serialise a parallel converter's data via shift registers, especially if opto-isolation is required. In such a case you may use the maximum serial clock of the DSP, which might be as high as 100 MHz on a Texas Instruments C6201 DSP, suitable for a 16 Bit 6.25 MHz data acquisition.If possible, use serial clocks and framesyncs generated by the DSP itself. This will provide a synchronisation of the multiple frequencies present in the system, and prevent beat frequencies and modulation products which might degrade your system's EMC.Data transfer from the serial port to the DSP memory can be accomplished by the DSP core itself in busy-polling or interrupt mode, or by DMA. DMA is the preferred choice, because it greatly relieves the DSP core. Please refer to section 2.3 for a comparison of these modes. Most DSPs feature separate internal bus-ses for their built-in peripherals, accessing them will not cause bus conflicts or stalls.© D.SignT 1999 Doc #1.062.2 Parallel InterfaceHigh-speed data acquisition usually requires the use of a parallel interface be-cause of the clock limitations of serial interfaces. Because modern DSPs typically provide only one external bus interface, care must be taken to avoid conflicts between the data acquisition and other peripherals or memories connected to the external bus.Figure 2-2 Parallel InterfaceLet's take a look at a typical scenario: A high-speed ADC operating at 20 MHz sampling frequency should be interfaced via the parallel external bus. Every 50 nsecs, a transfer must occur, otherwise data will be lost. Typical DSP bus cycles are about 30 nsecs, so reading the A/D converter (via DMA) should not present too much problems. However, if the acquisition buffer is located in external mem-ory, two memory accesses must be made in 50 nsecs ! It is a common practice to locate memory and peripherals in different memory banks to simplify address decoding and individually adjust the bus timing. However, often idle cycles are inserted by the DSP's external bus controller if a memory bank boundary is crossed. In the setup described above, an additional idle cycle before or after the converter is read would cause data acquisition to fail! If data acquisition needs not to be continuos, you may buffer the ADC data stream in a FIFO memory.In the setup described above, you must also make sure that no other peripheral will lock the external bus too long. Imagine a data transfer to a UART with 100 nsecs or more access time: at least one or two ADC samples will be lost!© D.SignT 1999 Doc #1.07If ADC data is acquired in busy-polling or interrupt mode, these calculations should be made for less demanding sampling frequencies as well. Even if a 1 MHz A/D converter must be regularly serviced by the DSP core, no other task or interrupt must run longer than 1 µsec. On a DSP with 20 nsecs instruction cycle time this is equal to processing 50 instructions. An interrupt service routine, e.g. to read data from a UART and perform flow control, might easily use that much instructions. If possible, assign the highest priority interrupt to the A/D converter and enable interrupt nesting, so the ADC may interrupt any other interrupt service currently executed.DMA transfers can help a lot to relieve constraints. If the converter is serviced by DMA, only the bus usage by memory and other peripherals must be observed.This directly leads to the question:2.3 Which maximum Data Acquisition Rate is possible ?The following examples assume an A/D converter connected either via a serial or parallel interface. Code samples are simplified because no instructions for buffer maintenance (range checking, wrap around in circular buffers) are included. Real-world code typically requires additional instructions, so the results should be regarded as best-case!2.3.1 InterruptsAn interrupt service routine (ISR) is used to read the converter and store the samples in an acquisition buffer. The interrupt is triggered by the ADC's conver-sion ready output.Written in C, the ISR code may be similar to:void ad_interrupt ( void ){buffer [index++] = *(int *)ADC_ADDRESS;}This looks pretty small and efficient, but take a look at what happens inside the DSP: If the interrupt occurs, first the code stored in the DSP execution pipeline© D.SignT 1999 Doc #1.08will be flushed, the current program counter is saved on the system stack, then the program counter will be loaded with a value from the interrupt vector table -the start address of the ISR. Next a context save is required, i.e. the current proc-essor status and all registers which are modified in the ISR must be pushed onto the stack. Then the ISR code is executed, the context is restored and the program counter is loaded with the value on top of the stack. The execution pipeline has to be filled again, finally processing is continued where it had been interrupted. Written in 'pseudo-code', the ISR will look like this:void ad_interrupt ( void ){save context (status register, registers used in ISR);load address register with 'buffer' start address;load address register with global constant 'ADC_ADDRESS'; load index register with global variable 'index';load general purpose register with data from ADC;store general purpose register at buffer+index;increment index;store the updated index register in global variable;restore context;return from interrupt;}As you can see, two address registers, an index register and a general purpose register are modified inside the ISR. Including a status register save and restore, context save and restore will require 5 instructions each. There is no chance for parallel execution except auto-incrementing the index, so the ISR will require 17 instructions. We still have to add several cycles for pipeline flush and re-fill and interrupt vector fetch, resulting in a typical runtime of approximately 24 cycles. A 'model' DSP with 20 nsecs instruction cycle will require 24 * 20nsecs = 480 nsecs. for interrupt processing, which will limit the reasonable data acquisition rate to 1M samples per second - at least there should remain some time to proc-ess the data.Remember, this is a best-case calculation! If index-boundaries have to be checked, execution time of the ISR will further increase. If any memory stall should occur, probably because the system stack and the program memory are located in the same memory bank, the execution time for context save and re-© D.SignT 1999 Doc #1.09store will double. The calculation above assumes an op-code fetch is possible in parallel with every load/store instruction.Also note that this result cannot be scaled linear with processor speed. A high-speed DSP like the Texas Instruments C6000 has an instruction cycle time of 5 nsecs only, but the execution pipeline is much longer, more cycles will be wasted by pipeline flush and re-fill. Also load instructions on the C6000 have a 4 cycle latency before the data is valid in the register. The C6000 compiler will try to interleave and re-arrange instructions to fill most of these 'delay-slots' with useful instructions, but this is rarely possible on such short program fragments like the ISR. Also more registers are required for interleaving, resulting in longer context save and restore time. If external memory is addressed, the execution time will be dominated by the external bus access time, which often needs to be much longer than 5 nsecs.Some C compilers do not track the register usage inside the ISR and perform a partial or complete context save and restore. An example is the Analog Devices C Compiler for the Sharc® series: You may select between three interrupt modes, the fastest one still requiring 30 cycles overhead. The Texas Instruments C Compiler do track the register usage and save and restore only those registers which are used inside the ISR. Make sure not to call any function inside the ISR, the compiler then can no longer track register usage and a complete context save and restore will be done.Speeding up interrupt processing by hand-coding in Assembler will not yield too much improvements unless you can reserve address and index registers for ex-clusive use in the ISR.Often some DSP instructions are not interruptable, like single cycle hardware loops. A typical instruction that may be assembled as a single cycle hardware loop by the compiler / optimiser may be:for (i=0; i<BUFFERSIZE; i++) buffer[i] = 0;Do not use interrupt driven data transfer for data acquisition above 1 MHz.Make sure the ADC interrupt has highest priority and nesting is enabled so it can interrupt a currently executed slow interrupt, e.g. a communications rou-tine.© D.SignT 1999 Doc #1.0102.3.2 Busy-PollingBusy-Polling techniques may be used for block-wise, non-continuos data acquisi-tion and simple algorithms like filters. The ADC 'conversion ready' signal is con-nected to an I/O port and sampled:void main ( void ){for (;;){while (!(*(int*)ADC_STATUS_PORT & CONV_READY_FLAG)) ;buffer [index++] = *(int*)ADC_ADDRESS;signal_processing ();}}The resulting pseudo-code is:void main ( void ){outer_loop:load address register with constant ADC_STATUS_PORT;inner loop:load general purpose register from ADC_STATUS PORT;calculate general purpose reg. AND bitmask CONV_READY;if result = zero jump inner_loop;load address register with constant ADC_ADDRESS;load address register with 'buffer' start address;load index register with global variable 'index';load general purpose register with data from ADC;store general purpose register at buffer+index;increment index;store the updated index register in global variable;call signal_processing;jump outer loop;}© D.SignT 1999 Doc #1.011The jump or branch instructions flush the pipeline and typically require 3 cycles. The resulting time spend inside the loops is 15 cycles (best case), corresponding to 300 nsecs on our model DSP. Assuming 500 nsecs are used for processing, data acquisition speed may be up to 1.25 MHz - not too much an improvement compared to interrupt processing. Some processors offer bit-test instructions or I/O ports whose state can be used as a condition for the branch instruction and thus save a few cycles. Note that these special instructions may not be supported by the C compiler and will require the use of inline assembly statements.Busy polling yields better results if data acquisition is done block-wise. In this case, addresses and indices remain in registers and do not require a re-load each loop. In this case, data acquisition is non-continuos: no data is acquired during signal-processing:void main ( void ){for (;;){/* Data Acquisition Loop */for (index=0; index<BLOCKSIZE; index++){while (!(*(int*)ADC_STATUS_PORT & CONV_READY_FLAG)) ; buffer [index] = *(int*)ADC_ADDRESS;}signal_processing();}}Because the address and index registers have to be loaded only once, the result-ing execution time of the for data acquisition loop is 7 cycles best-case only, suitable for 7 MHz acquisition speed on our model DSP.Busy-Polling is useful for block-wise, non-continuos data acquisition at high speeds. Make sure that no interrupts occur during polling and no DMA transfer locks the external bus too long if busy-polling is driven to it's limits.© D.SignT 1999 Doc #1.0122.3.3 DMAMost DSPs offer a DMA Controller that can be programmed to transfer data to and from memory to memory, or a built-in peripheral to memory without DSP core involvement. The DMA event is triggered by either an external DMA Request signal or by an internal status change, e.g. a serial port receiver full condition. The DMA controller can automatically increment addresses, sophisticated imple-mentations even maintain buffer structures like ping-pong or circular buffers on their own, some can even be used for sorting and two-dimensional DMA without CPU involvement. Auto-initialisation is required for these advanced features. Because the DSP core is not involved in data transfer, the only acquisition speed limitation is the maximum serial bit rate, respectively the external bus interface speed.The signal processing needs to be synchronised to the incoming data stream, this can be accomplished by using 'DMA complete' interrupts or polling of the DMA status. Best results are achieved in block mode. Because the DMA continues to acquire data during signal processing, data acquisition may be continuos:void main ( void ){DMA_source = ADC_ADDRESS;DMA_destination = buffer;DMA_source_increment = 0;DMA_destination increment = 1;DMA_count = BLOCKSIZE;DMA_auto_initialise = true;DMA_start;for (;;){while (! dma_complete) ;signal_processing ();}}© D.SignT 1999 Doc #1.013If the DMA controller lacks an auto-initialisation, this has to be performed by the DSP core:while (! dma_complete) ;DMA_destination = buffer;DMA_count = BLOCKSIZE;DMA_restart;The speed of DMA driven data acquisition is limited by the maximum serial bit rate, respectively the external bus speed only. A DSP with a 30 nsecs external bus cycle time may be used for data acquisition up to 30 MHz if the buffer is located in internal memory. If the DMA access conflicts with other external bus transactions, speed may be lower, e.g. 15 MHz if the buffer is located in ex-ternal memory too.Carefully check for bus idle cycles and possible bus conflicts to determine the maximum speed.As a result, use DMA wherever applicable. Even if acquisition speed is not too demanding, DMA will save plenty of DSP core processing time. This may allow to lower the system clock for power-saving, or give you some spare processing-time for future enhancements.© D.SignT 1999 Doc #1.0142.3.4 Still too slow ?Applications exist, where acquisition speeds above 30 MHz are desired. In these cases, the DSP will require some hardware assistance to manage data acquisi-tion. Several techniques exist:• You may include a hard-wired data acquisition and pre-processing, e.g. in a FPGA, to reduce the sampling frequency before the data is passed to the DSP for final processing.• In some cases a FIFO memory may be used to buffer the incoming data stream and allow block-read operations. This is especially true if idle cycles or bus conflicts may limit acquisition speed.• You may use an external ping-pong buffer, controlled by external hardware.The ADC is connected to bank 1 of the buffer, a counter generates incre-menting addresses to store the data. Bank 2 is connected to the DSP for processing. If processing and acquisition is complete, the banks are ex-changed. Crossbar switches or bus multiplexers can be used for bank-Figure 2-3 External Ping-Pong Buffer© D.SignT 1999 Doc #1.015• If multiple converters are used in synchronous sampling mode, you may con-nect them to a single address and different data bus lines. On a DSP with a32 bit external bus, you may connect a 16 bit converter to D0..D15 and an-other one to the same address, D16..D31. Reading both converters will be done in a single cycle. If acquisition speed of both converters may vary, you will need to generate a common 'conversion ready' signal by AND-ing both signals.• If a single converter with 16 bits or less is used, you may double the transfer rate if every second sample is latched and connected to the upper 16 bits of the DSP's 32 bit data bus. The DSP then reads sample(N) on D0..D15 and sample (N-1) on D16..D31 in a single bus cycle. The conversion ready signalFigure 2-4 Latched Samples• Typically, the converter's data width is less than the DSP data bus width. If the ADC uses 2's complement data, connecting it to the DSP's upper data bits will save additional shift or sign extension instructions, i.e. a 2's comple-ment 12 bit converter should be connected to D31..D20. The unconnected bits of the data bus will be undefined if the converter is read. To save masking operations, these bits may be driven low by an additional bus driver. Pull-Down resistors cannot be used because they must be as small as a few hun-dred ohms to un-load a 50pF bus capacitance fast enough, which will violate the DSP's bus driving capabilities.© D.SignT 1999 Doc #1.0163 Data OutputThe results of signal processing may be output via D/A converters, passed to other systems for further processing or visualisation, or control any kind of ma-chinery via I/O ports, field busses etc.For D/A converters, the same interface techniques, data transfer mechanisms and restrictions as described in the Data Acquisition section apply. In some cases, the DSP's built-in timer(s) can be used to generate a PWM output - a low cost D/A solution.Other output and communication interfaces are described in the following section.© D.SignT 1999 Doc #1.0174 Control InterfaceVery few DSP systems can operate without external control. Most applications require to pass process control information to the DSP and/or evaluate the results of signal processing. This may be accomplished by a direct connection of a user interface to the DSP (keypad, display), via serial and parallel connections (RS232, 422, SCSI), networks (Ethernet, CAN), or a direct bus interface to a micro-controller or host-system. The choice is usually dictated by the require-ments of data throughput, noise immunity and local distance of the systems.4.1 Host InterfaceIf a host-controller is located close to the DSP system, you may prefer to use a DSP with a built-in host interface. Two topologies exist: either the host interface uses it's own independent address, data and control signals, or the host may gain control over the DSP's external bus.The Texas Instruments C5000 and C6000 series for example use an independent host interface. The host can setup DMA transfers to and from DSP memory. Data is transferred via set of host port registers. The de-coupled interface ensures that a slow host will not slow down DSP operation.Analog Devices Sharc® processors include a bus arbitration logic which enables the host to gain control over the DSP external bus interface. The host may initiate DMA transfers from the DSP internal memory via a set of buffer registers or di-rectly access external peripherals and memories. Because the external bus con-trol is passed to the host, a slow host may lock the bus quite long, preventing the DSP from further operation if external memories or peripherals should be ac-cessed.If the DSP lacks a host interface you must attach a dual-ported memory or a bi-directional latch and control logic to the DSP external bus. An interface with indi-vidual transmit and receive buffers plus interrupt / DMA control can be imple-mented in a small CPLD. Compared to discrete logic, CPLD or FPGA imple-mentations will simplify matching the set-up and hold timing requirements to the DSP bus timing. The latch or dual ported memory de-couples the host and DSP busses and avoid bus locks caused by slow host processors. Transfers may be© D.SignT 1999 Doc #1.018DMA or interrupt driven, however, the host cannot initiate DMA transfers as it is possible on DSPs with a built-in host port. A communication protocol must be established to instruct the DSP to setup the requested transfers.If host communication is not too demanding, a serial interface may be used. Many micros feature a SPI interface which allows data transfers at several MBits/sec. SPI is based on a shift register with parallel load and straightforward to implement in a small CPLD or FPGA.4.2 Asynchronous CommunicationA UART can be connected to the DSP for standard asynchronous communica-tion. Typically the DSP bus timing and signals do not match the UART's bus interface, so some glue logic will be required. Only very few DSPs feature a built-in UART. The external UART is prone to cause problems with high speed data acquisition or extensive external memory accesses because of it's typically slow access times. Sophisticated UARTs are available with large receive and transmit FIFOs, DMA support and RTS/CTS or Xon/Xoff auto flow control. These features greatly reduce protocol overhead and may save valuable computation time.For less demanding requirements, the UART can be software-emulated by using the DSP I/O ports and timers. We have implemented this on a Texas Instruments TMS320C31 DSP. At 9600 baud, the UART functions require less than 3% of the DSP processing time, comparable to operating the DSP at 58 MHz instead of it's nominal 60 MHz.4.3 Bit-programmable I/O Portsare used for a direct connection of a user interface. Reading the status of switches, keypads or driving LEDs or LCD interfaces is a common use for I/O ports. Also reading the status of external peripherals, e.g. an ADC's conversion ready signal, is done via I/O. DSPs typically do not have sufficient I/O ports, so additional ports have to be conected to the external bus. Provided that address decoding is fast, latches and buffers may be accessed without wait states and will not cause too much conflicts with other external bus transactions.If a simple latch without read-back capability is used as an output port, a 'shadow' register must be maintained in the DSP memory to allow manipulation of individual bits.© D.SignT 1999 Doc #1.019CPLD and FPGA implementations of I/O ports make possible the integration of advanced features, e.g. bit-change interrupts, to relieve the DSP from control tasks. I/O port devices for micro controllers (PIO) are getting rare and suffer from slow interfaces. These devices should be used with care: the bus adaptation logic required to connect them to a fast DSP may be as costly as a direct implementa-tion of the I/O functionality in a CPLD/FPGA.4.4 SensorsMany signal processing applications must take into account the influence of envi-ronmental conditions like temperature to perform compensation or supervise limits. Time-keeping is also is often required. If these information cannot be supplied by a host system, suitable devices must be connected to the DSP. Often they are accessed in large time intervals only, because of slow variation of the measured quantity. Serial interfaces (SPI, Two-Wire, I²C) are preferable and commonly used. The serial interface can be either emulated via I/O ports in soft-ware or implemented in hardware, depending on how much time is left for soft-ware emulation. Other sensors might require a parallel bus interface. Again, ad-ditional glue logic and careful examination of bus conflicts is required.4.5 NetworksA variety of networks are used for communication in industrial and automotive systems. Mostly field busses like CAN have been used, but more and more Eth-ernet is making it's way. Most network controllers handle the physical layer and the lower protocol layers. They are typically designed for mass market applica-tions: ISA, PCI and PCMCIA/PC-Card busses. A few are designed to directly interface to commonly used micro controllers.None of these devices will fit to the external bus of a DSP without glue logic and timing adaptation. Slow bus cycles are common, which might cause bus locks.4.6 Other I/O InterfacesFireWire, USB, SCSI to name but a few, may also be used to communicate with other systems. Typically you will not find a DSP with these interfaces as a built-in peripheral, external controllers must be used. Bus adaptation and careful exami-nation of bus performance and conflicts is required.© D.SignT 1999 Doc #1.020。
- 1、下载文档前请自行甄别文档内容的完整性,平台不提供额外的编辑、内容补充、找答案等附加服务。
- 2、"仅部分预览"的文档,不可在线预览部分如存在完整性等问题,可反馈申请退款(可完整预览的文档不适用该条件!)。
- 3、如文档侵犯您的权益,请联系客服反馈,我们会尽快为您处理(人工客服工作时间:9:00-18:30)。
从系统的角度回答了“为什么采用DSP处理器”、“如何应
用DSP处理器进行开发和设计的工作” 等一系列基本问题。 使读者在学习具体内容前,对DSP应用技术先有一个全面、 概括的认识。
5
第3讲 DSP系统设计概述
2.1 DSP系统的基本构成
在进行DSP系统设计前,需要了解DSP系统的基本构成。典 型的DSP系统基本结构框图如图2-1所示。同一般的微处理器 应用系统类似,DSP应用系统除了DSP处理器之外,还必须 有能够与其他系统和器件连接的接口。
第3讲 DSP系统设计概述
2.2 DSP系统的设计开发过程
2.2.2 DSP系统的设计开发流程 4.系统集成和测试
软硬件设计、调试完成之后,进行系统集成。所谓系统集成 是利用DSP厂家提供的软件将软件程序生出固定的格式,写 入到DSP板上的EPROM或者Flash存储器中,代码固化后, DSP系统就可以脱离仿真器独立运行了。 DSP系统在可以独立运行之后,还应该继续进行一系列的系 统性能测试,评估系统的性能指标是否达到设计要求。在系 统测试的过程中,要反复检查系统的实时性、精度和稳定 性,如果达不到设计要求,就需要通过修改软件 ( 甚至调整 21 硬件)予以解决。
2.2.1 DSP系统设计开发前的准备工作 1.系统设计开发应具备的条件
(1)设计者知识储备
计者应当具备必需的数字信号处理知识,了解各种常用的数字信 号处理算法,并能够对信号处理算法的结果进行评估和比较。
设计者一方面要从整体上了解各个厂商各个系列的DSP处理器的 特点,另一方面还要及时跟踪和掌握DSP发展的新技术。 设计者对于外围电路芯片也应深入了解。
15
第3讲 DSP系统设计概述
2.2 DSP系统的设计开发过程
2.2.2 DSP系统的设计开发流程 2.确定算法并进行算法模拟
一般来说,为了实现系统的最终目标,需要对输入的信号进行适当的
处理,而不同的处理方法会导致不同的系统性能,因此要得到最佳的
DSP系统性能必须先确定最佳的处理方法。 确定算法并进行算法模拟阶段成为DSP实际系统设计中重要的一步,决
7
第3讲 DSP系统设计概述
2.1 DSP系统的基本构成
DSP处理器的输入是A/D转换后得到的时间离散的数字信号,根据系 统要求,DSP处理器对输入的数字信号按照特定的算法进行处理,这 是DSP系统的关键。最后,经过处理后的数字信号再经D/A转换器转 换为模拟信号,之后再进行平滑滤波就可得到连续的模拟信号。
Software Compatible
F2812: 业界最强大的控制专用DSP
Code security
128Kw Flash + 2Kw OTP 18Kw RAM 4Kw Boot ROM
TMX today, TMS Production mid 2003
Memory Sub-System
Event Mgr A Event Mgr B 12-Bit ADC
定了系统性能指标能否实现,系统以何种算法和结构应对需求。
实现过程:首先应对一个实时数字信号处理的任务选择一种方案和多 种算法,用算法仿真开发工具(如Matlab)进行算法模拟来验证算法能否 满足系统的性能指标,然后从多种信号处理算法中找出最佳算法。 16
第3讲 DSP系统设计概述
2.2 DSP系统的设计开发过程
通信设备 移动存贮
汽车电子
网络设备
网络
目录
2.1 DSP系统的基本构成
2.2 DSP系统的设计开发过程
2.3 主要DSP处理器 2.4 DSP系统的开发工具 2.5 典型的DSP应用系统 2.6 DSP最小系统 2.7 本章小结
4
第3讲 DSP系统设计概述
内容简介
本章从DSP系统设计的基础知识入手,介绍DSP系统的基本 构成、DSP系统的设计开发过程、DSP应用系统的开发工 具,并给出了典型的DSP应用系统示例。
将目标文件用链接器进行链接,得到可执行文件。
对可执行文件进行软件调试。检查运行结果是否正确。如果正确,进入 下一步;如果不正确,则返回第一步。软件调试借助DSP开发工具,如 软件模拟器(Simulator)、DSP开发系统或硬件仿真器等。 进行代码转换,将代码写入EPROM,并脱离仿真器运行程序,检查结 20 果是否正确。
2.2.2 DSP系统的设计开发流程 3.设计DSP应用系统(软硬件设计)
系统设计分硬件设计和软件设计两个方面同时进行。
硬件设计部分需要确定系统的硬件实现方案、完成器件的选 型、完成原理图设计(包括外围电路以及电源电路等)和印 刷电路板布线等,最后进行焊接调试。
软件设计部分主要是根据系统的要求和所选的DSP 处理器编 写相应的DSP程序并进行调试,这些程序可以采用汇编语 言、高级语言(如C 语言)、或采用两种语言(高级语言和 汇编语言)混合编程方法。
硬件设计又称为目标板设计,该设计需要在全面考虑应用的需 求分析、成本、体积、功耗核算等方面的基础上完成。
软件设计主要是指用DSP的汇编语言或者通用的高级语言(如C 语言)编写实现具体数字信号处理功能的程序。这两部分的设计 并非完全独立,而是需要协调综合考虑。
10
第3讲 DSP系统设计概述
2.2 DSP系统的设计开发过程
17
第3讲 DSP系统设计概述
2.2 DSP系统的设计开发过程
2.2.2 DSP系统的设计开发流程 3.设计DSP应用系统(软硬件设计)
(1) 硬件设计 硬件设计涉及较多的电路设计技术。由 DSP构成的电路一般包括以下类型的器 件:EPROM/F1ash、RAM、A/D、 D/A、同步/异步串口、电源模块、电平 转换器、FPGA、接口电路、仿真器接 口、时钟等。典型的DSP系统硬件设计 图2-3 DSP硬件设计流程 流程图如图2-3所示
22
C2000 DSP发展历程
High-Precision Control
High-endPS! Control Performance
F2812 F2810 176-LQFP 128-LQFP
F2812 179-u*BGA
Application specific versions
Peripheral Bus
XINTF
Fast program execution out of both RAM and Flash memory
110-120 MIPS with Flash Acceleration Technology 150 MIPS out of RAM for time-critical code Control Control Ports Peripherals
DSP处理器还提供了多种形式的串行通信接口,这些通信接口 能够方便地与一些串行设备进行通信。
9
第3讲 DSP系统设计概述
2.2 DSP系统的设计开发过程
DSP系统的设计开发过程可以分为系统需求分析、算法模拟、 DSP处理器选型、软硬件设计、系统集成和测试5个阶段。
软硬件设计包括软件设计和硬件设计。
8
第3讲 DSP系统设计概述
2.1 DSP系统的基本构成
除了处理从外部信号源输入的信号外,DSP还需要处理从主机 或用户控制接口输入的事件。 主机接口是一个并行接口,可以用来与一个主处理器或一个主 器件进行连接,完成DSP处理器和主机之间的信号交换。
用户控制接口完成DSP处理器与用户需求之间的交换。
18
第3讲 DSP系统设计概述
2.2 DSP系统的设计开发过程
2.2.2 DSP系统的设计开发流程 3.设计DSP应用系统(软硬件设计)
(1) 硬件设计 硬件设计的过程可分为以下5个阶段: 确定硬件实现方案 器件的选择
原理图设计
PCB板设计 硬件调试
19
第3讲 DSP系统设计概述
2.2.2 DSP系统的设计开发流程 1.定义系统性能指标
在进行DSP系统设计之前,首先要根据 DSP系统的需求,明
确设计任务,定义系统的技术性能指标。
技术性能指标,包括系统的采样频率和实时处理性能、存储
器容量、系统的精度、应用环境、体积、重量、功耗、可靠
性、可维护性以及成本等要求,它们通常可用数据流程图、 数学运算序列、正式的符号或自然语言来描述。
12
第3讲 DSP系统设计概述
2.2 DSP系统的设计开发过程
2.2.1 DSP系统设计开发前的准备工作 2.初学者如何着手DSP系统开发
当初学者确定用DSP处理器进行系统设计时,需要准备的最 基本的设备包括一台装有 Windows 操作系统的 PC 机、一套 DSP开发板、一个硬件仿真器以及基本的软件开发工具。 DSP系统设计中,初学者有了这些最基本设备的支持,硬件 上借助 DSP 厂商提供的 DSP开发板,软件上借助 DSP 厂商提 供的基本软件开发工具,只需编写一些简单的应用程序即可 完成DSP系统的设计。
对于高速的数字、模拟电路设计,设计者应具备相应的知识和经 验。在设计中,应采取一定的抗干扰措施。
11
第3讲 DSP系统设计概述
2.2 DSP系统的设计开发过程
2.2.1 DSP系统设计开发前的准备工作 1.系统设计开发应具备的条件
(2)软件环境和硬件设备支持
DSP系统设计开发的软件环境应该包括针对特定DSP处理器的 编译器、汇编器、链接器、软件模拟器、在线仿真软件、固化 代码生成程序、库管理程序等可执行文件,还应包括基本的算 法或函数库、C语言库、C 头文件等。 硬件设备包括DSP仿真器、示波器和逻辑分析仪。其中,最主 要的就是DSP仿真器,各DSP厂家为不同的DSP准备了不同的 仿真器。
第3讲 DSP系统设计概述
2.3 主要DSP处理器